LTE发射机ACLR性能地测量技术
中国移动TD-LTE实验室测试RF规范

中国移动通信企业标准QB-╳╳-╳╳╳-╳╳╳╳T D-L T E无线子系统射频测试规范T D-L T E R A N S u b-s y s t e m T e s tS p e c i f i c a t i o n f o r R F d i v i s i o n版本号:1.1.0╳╳╳╳-╳╳-╳╳发布╳╳╳╳-╳╳-╳╳实施中国移动通信集团公司发布<测试规范定义>测试规范是对网络设备/网络接口协议/设备性能进行的测试的依据,力图对该设备的功能,接口,协议,性能等各方面进行全面的测试。
该类技术文件应具有如下特点:1、全面性该类规范应该在其规定的测试范围内的进行全面的测试,以便反映该设备的是否真正正确的实现了功能/协议,以便完成对该设备的评价。
2、正确性测试规范作为鉴定设备的正确性的依据。
其表述的内容必须首先是正确的。
判断正确与否的测试结果必须是可以正确得到的,也是设备本身能够完成和必须完成的。
3、容错性测试规范必须对发生错误情况下设备的反馈进行详细的测试。
测试项目必须全面包括各种异常情况。
4、权威性该类规范是集团公司在测试和检验方面的重要文件,应该观点明确,测试项目全面,论述过程不应体现在正文中,可以根据情况在附件或编制说明中体现;在用辞上注意规范的强制性,不应使用建议性的语气。
所有检验结果都必须是确定的。
5、强可操作性该类规范是实际指导测试的文件,因此要具有强可操作性。
该规范直接为技术人员所利用,相关人员应该可以按照规范的规定直接进行实际测试。
<使用范围>中国移动通信集团内部,外部,用于指导集团公司和省公司进行网络实施、新业务开展时的设备测试和验收。
<与其他规范之间关系>在业务规范,总体技术要求,设备规范、接口规范基础上完成,是进行组建一个网络或者业务系统的设备的验收性指导性规范。
<主要内容>主要包括测试环境,测试配置,测试工具及测试方法的描述,设备的常规测试、功能测试、接口测试、协议测试、质量指标测试(性能测试)、计费结算功能测试、业务测试、网络管理、人机界面测试、可靠性测试、网络安全测试等等,目的是对在规定的范围内,对设备进行详尽的测试。
2021移动LTE认证考试题库及答案13
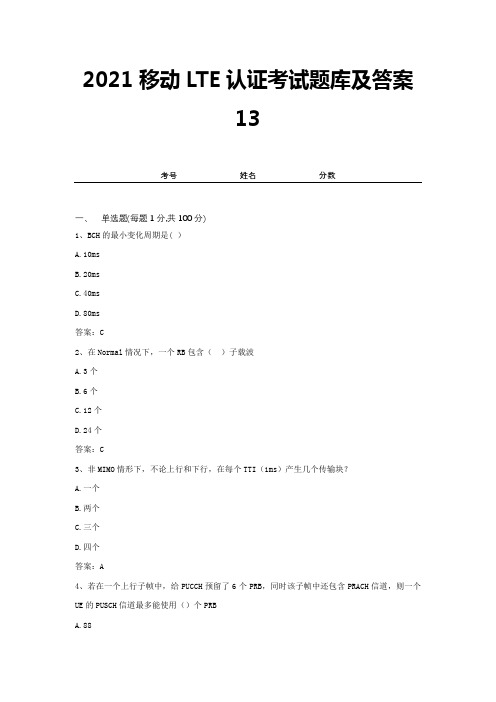
2021移动LTE认证考试题库及答案13考号姓名分数一、单选题(每题1分,共100分)1、BCH的最小变化周期是( )A.10msB.20msC.40msD.80ms答案:C2、在Normal情况下,一个RB包含()子载波A.3个B.6个C.12个D.24个答案:C3、非MIMO情形下,不论上行和下行,在每个TTI(1ms)产生几个传输块?A.一个B.两个C.三个D.四个答案:A4、若在一个上行子帧中,给PUCCH预留了6个PRB,同时该子帧中还包含PRACH信道,则一个UE的PUSCH信道最多能使用()个PRBA.88B.94C.81D.80答案:A5、PBCH共占用()个RB资源。
()A.6B.7C.8D.9答案:A6、UTPRc单板-TDL不能支持()无线制式A.GSMB.TDC.LTED.CDMA答案:D7、S1接口的用户面终止在什么上?A.SGWB.MMEC.MMHD.SAW答案:A8、RSSI单位是什么A.dBmB.dBC.dBiD.dBd答案:A9、下载截图时需要A.不做要求B.要保证Radio Parameters和HooNetMeter的完整C.要保证Radio Parameters完整D.保证HooNetMeter完整答案:B10、双通道室分单极化天线布放在狭长走廊场景,建议布放天线间距小于()个波长(65cm),且尽量使天线的排列方向与走廊方向垂直,以降低天线相关性。
A.3B.4C.5D.6答案:D11、LTE系统中,每个小区用于随机接入的码是preamble码,一共()个A.32个B.64个C.128个D.256个答案:B12、目前EMS中使用的统一网管平台为:A.UEP1XB.UEP2XC.UEP3XD.UEP4X答案:D13、地铁覆盖中,各运营商通过()将信号合路到泄漏电缆上A.POIB.耦合器C.功分器D.滤波器答案:A14、当使用不同循环时间移位的UE数量()可支持的循环时间移位数量时,分配具有最大可能间距的循环时间移位是有利的,在LTE中也得到支持。
LTE干扰研究方法
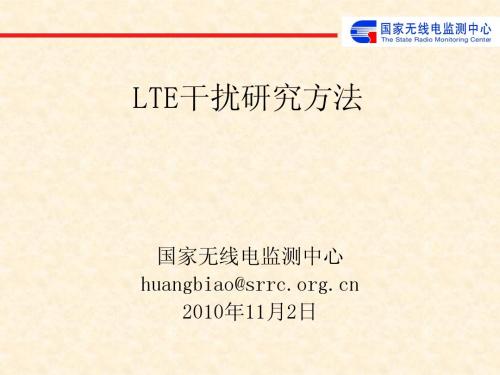
网络拓扑结构
• 采用19小区六边形 宏蜂窝扇区化模型, 每小区分3个扇区, 小区半径R=ISD/3 (ISD为站间距), 采用Wrap-around技 术消除边缘效应
功率控制算法
γ PL P = Pmax × min 1, max Rmin , t PLx−ile
上行干扰计算
SINRi = P ,i ⋅ Gk ,i ⋅ PLk ,i k
m≠k m=1
∑P
N
⋅ Gm,i,k ⋅ PLintra + ∑∑ P , j ⋅ Gn, j ,k ⋅ PLinter ⋅ ACIR + N0 m,i n m,i ,k n , j ,k
n=1 j =1
N
Nu
• SINRi表示基站k在信道i上的接收信噪比; • Pk, i表示占用信道i的用户的发射功率; • Pm, i表示本系统扇区m中占用信道i的用户的发射功率; • Pn, j表示外系统扇区n内用户j的发射功率; • Gm, i, k表示本系统扇区m中占用信道i的用户与基站k之间的收发天线增 益之和; • Gn, j, k表示外系统扇区n中用户j与基站k之间的收发天线增益之和; • Nu表示干扰系统每扇区上行用户数。
• 综合方法
– 综合利用确定性分析和系统仿真方法最终得出系统共存所需的隔 离度
蒙特卡罗仿真
• 对基站和移动台的发射功率、基站的负载等情况进行仿真,将整个系统的运 转区间划分为若干个间隔,每两个间隔之间为一个快照(Snap-Shot)取样 时刻,将所有快照时刻的取样结果进行记录,用统计方法加以分析,产生所 需要的结果。 • 每个快照中,在整个系统覆盖范围内随机均匀分布移动台,使得每个小区内 用户数相同。 • 对所有小区进行性能仿真。对于每个小区,首先根据调度准则选择一个被调 度的UE,从未被调度的RB中取出10个RB(以10MHz带宽为例)分配给该 UE,并将这10个RB标记为“已调度”。然后,根据相应的功率控制算法设 置UE的发射功率。重复上述过程,直到所有RB都标记为“已调度”。 • 对每一个UE计算实际的系统内/系统间干扰,以得到实际的C/(I+N)和比特率。 在计算系统内干扰时,只考虑与该UE占用相同RB编号的外小区UE;在计算 系统间干扰时,要考虑将接收到的外系统干扰信号进行邻频衰减。 • 收集每个小区的数据,并进行统计平均。
LTE band 1 发射功率指标要求
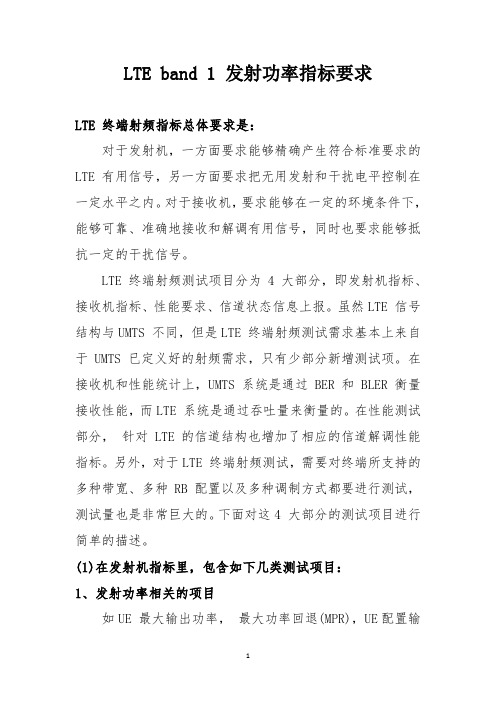
LTE band 1 发射功率指标要求LTE 终端射频指标总体要求是:对于发射机,一方面要求能够精确产生符合标准要求的LTE 有用信号,另一方面要求把无用发射和干扰电平控制在一定水平之内。
对于接收机,要求能够在一定的环境条件下,能够可靠、准确地接收和解调有用信号,同时也要求能够抵抗一定的干扰信号。
LTE 终端射频测试项目分为4 大部分,即发射机指标、接收机指标、性能要求、信道状态信息上报。
虽然LTE 信号结构与UMTS 不同,但是LTE 终端射频测试需求基本上来自于UMTS 已定义好的射频需求,只有少部分新增测试项。
在接收机和性能统计上,UMTS 系统是通过BER 和BLER 衡量接收性能,而LTE 系统是通过吞吐量来衡量的。
在性能测试部分,针对LTE的信道结构也增加了相应的信道解调性能指标。
另外,对于LTE 终端射频测试,需要对终端所支持的多种带宽、多种RB 配置以及多种调制方式都要进行测试,测试量也是非常巨大的。
下面对这4 大部分的测试项目进行简单的描述。
(1)在发射机指标里,包含如下几类测试项目:1、发射功率相关的项目如UE 最大输出功率,最大功率回退(MPR),UE配置输出功率等。
这些测试项目,主要是考察终端的发射功率是否符合标准要求。
如果终端最大发射功率过大,会对其他信道或系统造成干扰,最大发射功率过小会造成系统覆盖范围减少。
最大功率回退是新增测试项,后面会做详细分析。
2、输出功率动态范围如最小输出功率、发射关断功率、开关时间模板等。
这些测试项目,主要是考察终端的输出功率范围是否符合标准要求。
如果最小输出功率以及关断功率过大,就会对其他终端和系统造成干扰。
开关时间模板验证终端能否准确地打开或者关闭其发射机,否则会对其他信道造成干扰或者增加上行信道的发射误差。
3、功率控制如绝对功率控制容限、相对功率控制容限等。
功率控制的目的是限制终端的干扰电平和补偿信道衰落,这部分测试主要是验证终端能否正确的设置其发射功率,并且发射功率在一定的容限范围之内。
LTE_ACLR

Keysight TechnologiesMeasuring ACLR Performancein LTE TransmittersApplication NoteIntroductionAs wireless service providers push for more bandwidth to deliver IP services to more users, LTE hasemerged as a next-generation cellular technology with great potential to enhance current deployments of 3GPP networks and to enable signiicant new service opportunities. However, LTE’s complex, evolved archi-tecture introduces new challenges in designing and testing network and user equipment. The commercial success of LTE will depend in part on the ability of all devices to work as speciied. One of the particular challenges at the air interface will be power management during signal transmission.In a digital communication system such as LTE, the power that leaks from a transmitted signal into adjacent channels can interfere with transmissions in the neighboring channels and impair system performance. The adjacent channel leakage-power ratio (ACLR) test veriies that system transmitters are performing within speciied limits. This critical yet complex transmitter test can be made quickly and accurately using modern signal analyzers such as the Keysight Technologies, Inc. X-Series (PXA/MXA/EXA) signal analyzers with LTE measurement software and signal generators such as the Keysight MXG signal generator with LTEsignal creation software.Challenges of LTE transmitter designLTE product development is underway, and RF engineers are tackling the many design and measurement challenges this complex technology presents. LTE requires support for six channel bandwidths (from 1.4 to 20 MHz), different transmission schemes for the downlink and the uplink (OFDMA and SC-FDMA), two transmission modes (FDD and TDD), and multiple antenna techniques (MIMO spatial multiplexing, diversity, beamsteering). As a result of LTE’s lexible transmission schemes, the physical channel coniguration has a large impact on RF performance—much greater than in current CDMA-based systems. With performance targets set exceptionally high for LTE, engineers have to make careful design tradeoffs to cover each criti-cal part of the radio transmitter chain.One important aspect of transmitter design is the need to minimize unwanted emissions. Because LTE will be deployed in the same frequency bands as W-CDMA and other legacy cellular technologies, the 3GPP speciications regulate emissions to minimize interference and ensure compatibility between the different radio systems. The primary concern is control of spurious emissions, which can occur at any frequency. In this respect LTE is similar to other radio systems. However, new challenges arise at the band edges, where the transmitted signal must comply with rigorous power leakage requirements. With LTE supporting chan-nel bandwidths up to 20 MHz, and with many bands too narrow to support more than a few channels, a large proportion of the LTE channels will be at the edge of the band.Controlling transmitter performance at the edge of the band requires a design with iltering to attenuate out-of-band emissions without affecting in-channel performance. Factors such as cost, power eficiencies, physical size, and location in the transmitter block diagram are also important considerations. Ultimately the LTE transmitter must meet all speciied limits for unwanted emissions, including limits on the amount of power that leaks into adjacent channels, as deined by ACLR.ACLR test requirements ACLR is a key transmitter characteristic included in the LTE RF transmitterconformance tests (Table 1). These tests verify that minimum requirements arebeing met in the base station (eNB) and user equipment (UE). Most of the LTEconformance tests for out-of-band emissions are similar in scope and purposeto those for W-CDMA and should look familiar. However, while W-CDMA speci-fies a root-raised cosine (RRC) filter for making transmitter measurements, noequivalent filter is defined for LTE. Thus different filter implementations canbe used for LTE transmitter testing to optimize either in-channel performance,resulting in improved error vector magnitude, or out-of-channel performance,resulting in better adjacent channel power characteristics.Table 1. Conformance tests for RF transmitters (from 3GPP TS 36.141 [1] and 36.521-1 [2]) Array ArrayGiven the extensive number of complex transmitter configurations that canbe used to test transmitter performance, LTE specifies a series of downlinksignal configurations known as E-UTRA test models (E-TM) for testing theeNB. These test models are grouped into three classes: E-TM1, E-TM2, andE-TM3. The first and third classes are further subdivided into E-TM1.1, E-TM1.2,E-TM3.1, E-TM3.2, and E-TM3.3 (Table 2). Note that the “E” in E-UTRA stands for“enhanced” and designates LTE UMTS terrestrial radio access, whereas UTRAwithout the “E” refers to W-CDMA.ACLR test requirements (continued)For UE testing, transmitter tests are carried out using the reference measurement channels (RMC) specified for eNB receiver testing. The ACLR requirement for the UE is not as stringent as it is for the eNB, so our focus will be on the latter.Table 2. E-UTRA test models (from 3GPP TS 36.141 [1])The 3GPP specifications for LTE define ACLR as the ratio of the filtered mean power centered on the assigned channel frequency to the filtered mean power centered on an adjacent channel frequency. Minimum ACLR conformance requirements for the eNB are given for two scenarios: for adjacent E-UTRA (LTE) channel carriers of the same bandwidth, E-UTRAACLR1, and for the UTRA (W-CDMA) adjacent and alternate channel carriers, UTRAACLR1and UTRAACLR2, respectively.Different limits and measurement filters are specified for E-UTRA and UTRA adja-cent channels, and are provided for both paired spectrum (FDD) operation and unpaired spectrum (TDD) operation. The E-UTRA channels are measured using a square measurement filter, while UTRA channels are measured using an RRC filter with a roll-off factor of = 0.22 and a bandwidth equal to the chip rate, whichis 3.84 MHz in the example of paired spectrum operation shown in Figure 1.Figure 1. Measurement filters forACLR measurements ACLR limits definedfor adjacent E-UTRA carriers ACLR limits definedfor adjacentUTRA carriersACLR test requirements (continued)ACLR test requirements for the eNB including paired and unpaired spectrum operation are summarized in Table 3. As of the September 2009 specification release, the ACLR test cases for the UE were not fully complete. However, the UE test procedure is essentially the same as that used for the base station.Table 3. ACLR base station conformance test requirements (from 3GPP TS 36.141 6.2 [1]). Note that the specification defines the minimum requirement plus the test tolerance (TT)). * Relative limits are 44.2 dB = 45 dB min requirement + 0.8 dB TT. Both Absolute and Relative limitsare provided. Whichever is less stringent is to be used for the conformance requirement. Sophisticated signal evaluation tools are available for making complex LTE measure-ments quickly and accurately. Power measurements including ACLR generally are made using a spectrum or signal analyzer, and the required test signals are built using a signal generator. In the following examples, Keysight’s PC-based Signal Studio application connected to an MXG signal generator is used to build the standards-compliant E-TM signal required for transmitter testing. The output signal is connected to the RF input of an Keysight X-Series signal analyzer running N9080A LTE measure-ment application, which is used for signal analysis. This equipment setup follows the simple block diagram provided in the 3GPP LTE specifications (Figure 2). Although the measurement process described here is for FDD operation, the process for TDD operation is similar.According to the specifications, the carrier frequency must be set within a frequency band supported by the base station under test, and ACLR must be measured for frequency offsets on both sides of the channel frequency, as specified for paired or unpaired spectrum operation (T able 3). The test is performed first using a transmitted signal of type E-TM1.1, in which all of the PDSCH resource blocks have the same power, and then again using E-TM1.2, in which power boosting and deboosting are used. The E-TM1.2 configuration is useful because it simulates multiple users whose devices are operating at different power levels. This scenario results in a higher crest factor, which makes it more difficult to amplify the signal without creating additional,unwanted spectral content—i.e., ACLR.Spectrum Bands Adjacent Carrier Limits (Min req + TT) *PairedSpectrumCategory AE-UTRA 44.2 dB or -13 dBm/MHzUTRA 44.2 dB or -13 dBm/MHz PairedSpectrumCategory BE-UTRA 44.2 dB or -15 dBm/MHzUTRA 44.2 dB or -15 dBm/MHzUnpairedspectrumCategory AE-UTRA (LTE) 44.2 dB or -13 dBm/MHz1.28 Mcps UTRA 44.2 dB or -13 dBm/MHz3.84 Mcps UTRA 44.2 dB or -13 dBm/MHz7.82 Mcps UTRA 44.2 dB or -13 dBm/MHzUnpairedspectrumCategory BE-UTRA (LTE) 44.2 dB or -15 dBm/MHz1.28 Mcps UTRA 44.2 dB or -15 dBm/MHz3.84 Mcps UTRA 44.2 dB or -15 dBm/MHz7.82 Mcps UTRA 44.2 dB or -15 dBm/MHzSetting up the ACLR testFigure 2. Measurement equipment setup (3GPP TS 36.141 [1] Annex I, Figure I.1-1)Setting up the ACLR test (continued)In this ACLR measurement example, Signal Studio is set up to generate a standards-compliant E-TM1.2 test signal. The frequency is set to 2.11 GHz, a frequency that is in several of the major downlink operating bands specified for LTE. The output signal amplitude—an important consideration in determining ACLR performance—is set to -10 dBm. A 5 MHz channel bandwidth is selected from the range that extends from 1.4 to 20 MHz.Figure 3 shows the eNB setup with Transport Channel selected. A graph of the resource allocation blocks for the test signal appears at the bottom. The Y axis indicates frequency or resource blocks, while the X axis indicates slots or time. The different colors correspond to different channels, with the white areas representing Channel 1 and the pink areas Channel 2. Both are downlink shared channels, of interest in this measurement. The other colors represent synchroni-zation channels, reference signals, etc.Figure 3. Resource allocation blocks (at bottom) for E-TM1.2 test signalSelecting Channel 1 shows the output power level to be at -4.3 dB, so the channel power has been deboosted. The output power of Channel 2 has been boosted and is set at 3 dB (Figure 4). A complex array of power boosting and deboosting options can be set for the different resource blocks from resource block allocation graph. The resulting composite signal will have a higher peak-to-average ratio than a single channel in which all blocks are at the same power level. Amplifying a boosted signal such as this can be difficult, as noted earlier. Without sufficient back-off in the power amplifier, clipping may result.Figure 4. Boosted output power in Channel 2Setting up the ACLR test(continued)The test signal is now generated using the Signal Studio software. Because Signal Studio is PC-based, it can be run from the PC-based X-Series signal ana-lyzer. The waveform in this case is created on the desktop of the signal analyzer and then downloaded to the signal generator via LAN or GPIB. The RF output of the signal generator is connected to the RF input of the signal analyzer, where the ACLR performance is measured using swept spectrum analysis. In this example, the signal analyzer is in LTE mode with a center frequency of 2.11 GHz and the ACP measurement selected. At this point it is possible to make a quick, one-button ACLR measurement according to the LTE standard by recalling the appropriate parameters and test limits from a list of available choices in the LTE application. These choices include options for paired and unpaired spectrum, Category A or Category B limits (as defined in ITU-R SM.329), and type of carrier in the adjacent and alternate channels—E-UTRA (LTE), UTRA (W-CDMA), or TD-SCDMA.Recall that for FDD operation, LTE defines two different methods of making ACLR measurements. In Figure 5, the upper graph shows the case in which E-UTRA (LTE) is used at the center and offset frequencies. The lower graph shows LTE at the center frequency and UTRA (W-CDMA) at the adjacent and alternate offsets.Figure 5. Two specified methods of ACLR measurementIn Figure 6 the measurement result shows the E-UTRA adjacent and alternate offset channels. For this measurement a 5 MHz carrier is selected; however, the measure-ment noise bandwidth is 4.515 MHz, because the downlink contains 301 subcarriers. The first offset (A) is at 5 MHz, with an integration bandwidth of 4.515 MHz. The second offset (B) is at 10 MHz with the same integration bandwidth.Figure 6. ACLR measurement result using Keysight X-Series analyzer before optimization This one-button measurement gives a very quick, usable ACLR measurementaccording to the LTE standard. While the result in Figure 6 of about -62 dBc is good, the analyzer settings can be optimized to get even better performance. Four ways to further improve the measurement results are (1) optimize the signal level at the mixer; (2) change the resolution bandwidth filter; (3) turn on noise correction; and (4) use a different measurement methodology called filtered integration bandwidth.To optimize the signal level at the input mixer, the attenuator is adjusted for minimal clipping. The X-Series signal analyzer will automatically select an attenuation value based on the current measured signal value. This automated technique provides a good starting point for achieving optimal measurement range. Signal analyzers such as the X-Series, which have both electronic and mechanical attenuators, can use the two in combination to optimize performance. In such cases the mechanical attenuator can be adjusted slightly to get even better results of about 1 or 2 dB.Next the resolution bandwidth can be lowered by pressing the bandwidth filter key. Note that sweep time increases as the resolution bandwidth is lowered. For example, with the MXA signal analyzer, sweep time at 30 kHz is 676.3 ms. At a lower 10 kHz RBW, the sweep time is about 6 seconds. The slower sweep time reduces variance in the measurement, but reduces measurement speed.Another step is to turn on noise correction. The analyzer then takes one sweep to measure its internal noise floor at the current center frequency, and in subse-quent sweeps subtracts that internal noise floor from the measurement result. This technique substantially improves ACLR, in some cases by up to 5 dB.Setting up the ACLR test(continued)Optimizing theanalyzer settingsOptimizing the analyzer settings (continued)Changing the measurement method is a fourth way to optimize the analyzer. In this case the default measurement method (integration bandwidth or IBW) is changed to the filtered IBW method. Filtered IBW uses a sharp, steep cutoff filter. This technique does degrade the absolute accuracy of the power measurement result, but it does not degrade the relative power accuracy, and ACLR is a relative power measurement. Therefore, filtered IBW does not degrade the ACLR result. Using these techniques in combination, an Keysight’s X-series analyzer can optimize the ACLR measurement automatically for performance versus speed via the analyzer’s embedded LTE application. The result for a typical ACLR mea-surement is improved by up to 10 dB or more. Figure 7 shows an 11 dB ACLR improvement after optimization (compared to Figure 6) using the embedded LTE application. For measurement scenarios requiring the maximum performance, the analyzer settings can be further adjusted.Figure 7. ACLR measurement result using Keysight X-series analyzer after optimization Standards-compliant spectrum measurements such as ACLR are invaluable for RF engineers developing the next generation radio systems. With LTE, however, these measurements are complicated by factors such as variations in the band-width of adjacent channels, choice of transmission filter, and interaction of RF variables between channels of different bandwidth and different susceptibility to interference. A practical solution is to use a spectrum or signal analyzer with a standards-specific measurement application. This combination can reduce error in complex measurements, automatically configuring limit tables and specifiedtest setups and ensuring measurement repeatability.ConclusionAcronyms3GPP 3rd Generation Partnership ProjectACLR Adjacent channel leakage-power ratioA-MPR Additional maximum power reductionCDMA Code division multiple accesseNB Evolved node BE-TM E-UTRA test modelE-UTRA Evolved universal terrestrial radio accessEVM Error vector magnitudeFDD Frequency division duplexGPIB General purpose interface busIBW Integration bandwidthLAN Local area networkLTE Long term evolutionMPR Maximum power reductionMIMO Multiple input multiple outputOFDMA Orthogonal frequency division multiple accessPRACH Physical random access channelQAM Quadrature amplitude modulationQPSK Quadrature phase-shift keyingRB Resource blockRBW Resolution bandwidthRE Resource elementRF Radio frequencyRRC Root-raised cosineSC-FDMA Single carrier frequency division multiple accessSRS Sounding reference signalTDD Time division duplexTD-SCDMA Time domain synchronous code division multiple accessUE User equipmentUMTS Universal mobile telecommunications systemUTRA Universal terrestrial radio accessW-CDMA Wideband code division multiple access11ReferencesMore Information[1] 3GPP TS 36.141 V8.4.0 (2009-09) Base Station (BS) Conformance Testing [2] 3GPP TS 36.521-1 V8.3.1 (2009-09) User Equipment (UE) Conformance Specification; Radio Transmission and Reception Part 1: Conformance T estingFor more information about the 3GPP, visit the 3GPP home page /3GPP specifications home page/specs/specs.htm 3GPP Series 36 (LTE) specifications/ftp/Specs/archive/36_seriesFor more information about Keysight design and test products for LTE visit /find/lteKeysight LTE application notes and technical overviews:3GPP Long T erm Evolution: System Overview, Product Development, and Test Challenges: 5989-8139EN LTE Component T est: 5990-5149ENMIMO in LTE Operation and Measurement—Excerpts on LTE T est: 5990-4760EN MIMO Performance and Condition Number in LTE T est: 5990-4759ENN9080A & N9082A LTE Modulation Analysis Measurement Application Technical Overview: 5989-6537ENLearn more about LTE and its measurements in the new book written by 30 LTE experts:LTE and the Evolution to 4G Wireless Design and Measurement Challenges/find/ltebookFor more information on KeysightTechnologies’ products, applications or services, please contact your local Keysight office. The complete list is available at:/find/contactus Americas Canada (877) 894 4414Brazil 55 11 3351 7010Mexico001 800 254 2440United States (800) 829 4444Asia Paciic Australia 1 800 629 485China800 810 0189Hong Kong 800 938 693India 1 800 112 929Japan 0120 (421) 345Korea 080 769 0800Malaysia 1 800 888 848Singapore 1 800 375 8100Taiwan0800 047 866Other AP Countries (65) 6375 8100Europe & Middle East Austria 0800 001122Belgium 0800 58580Finland 0800 523252France 0805 980333Germany 0800 6270999Ireland 1800 832700Israel 1 809 343051Italy800 599100Luxembourg +32 800 58580Netherlands 0800 0233200Russia 8800 5009286Spain 0800 000154Sweden 0200 882255Switzerland0800 805353Opt. 1 (DE)Opt. 2 (FR)Opt. 3 (IT)United Kingdom0800 0260637For other unlisted countries:/find/contactus(BP-07-10-14)This information is subject to change without notice.© Keysight Technologies, 2009-2014Published in USA, July 31, 20145990-5089EN12 | Keysight | Measuring ACLR Performance in LTE Transmitters - Application NoteLAN eXtensions for Instruments puts the power of Ethernet and the Web inside your test systems. Keysight is a founding member of the LXI consortium.Keysight Assurance Plans/find/AssurancePlansUp to five years of protection and no budgetary surprises to ensure your instruments are operating to specification so you can rely on accurate measurements.Keysight Channel Partners/find/channelpartnersGet the best of both worlds: Keysight’s measurement expertise and product breadth, combined with channel partner convenience./find/lte。
TD-LTE基站射频测试步骤详解

TD-L TE基站射频测试操作说明第一部分TX测试一、TX测试连接图RRH衰减器频谱分析仪BBUTrigger ReferenceNote: 衰减为输出功率2.5倍以上,此处选择30dB。
10MH 参考线时钟线reference接BBU针孔,10毫秒trigger接B板最右边网口。
二、仪表等附件内容频谱分析仪(Agilent MXA Signal Analyzer N9020A,10Hz~13.6GHz), 30dB衰减,System DC Power 7Supply (Agilent N5747A/60V/12.5A/750W),VGA信号源。
LTE NEM,Secure CRT。
三、NEM操作系统配置四、频谱仪选键设置Step 1. 选择LTE模式,Press Mode, LTE TDD.Step 2. 频点设置FREQ ChannelStep 3. 频点补偿设置Input/Output, External Gain, BTSStep 4. 下行模式设置Mode Setup, Radio, Direction to be Downlink.Step 5. 链路配置设Mode Setup, Radio, ULDLAlloc, Config 3;DW/GP/Up Len, More, Config 8.Step 6. 时间门限设置Sweep/control, Gate, Gate Delay=5ms, Gate Length=6.8msStep 7. 带宽设置Mode setup, Prest To Standard=10/20MHzStep 8. 触发方式设置Trigger, External 1/2配置完后频谱分析仪显示如下:五、下行发射项测试操作步骤1. 输出功率Output Power测试项选择Meas---Channel power测试模式E-TM1.1Note: 查看Channel Power值,(10W标准值40dBm)。
中国移动TD-LTE一体化皮基站设备必达标技术要求
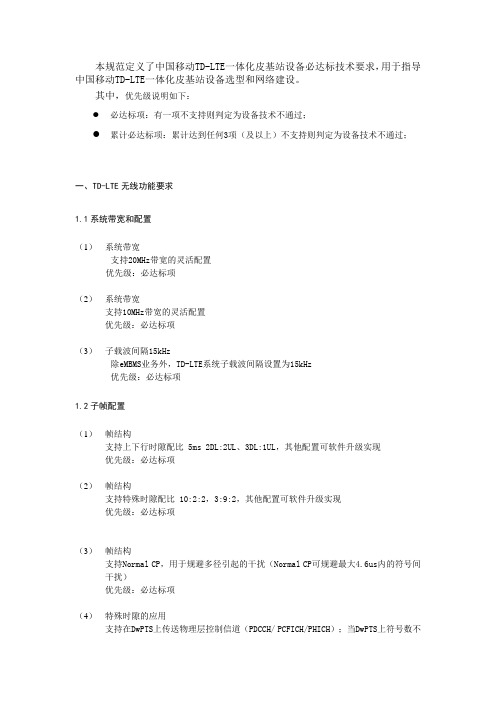
本规范定义了中国移动TD-LTE一体化皮基站设备必达标技术要求,用于指导中国移动TD-LTE一体化皮基站设备选型和网络建设。
其中,优先级说明如下:●必达标项:有一项不支持则判定为设备技术不通过;●累计必达标项:累计达到任何3项(及以上)不支持则判定为设备技术不通过;一、TD-LTE无线功能要求1.1系统带宽和配置(1)系统带宽支持20MHz带宽的灵活配置优先级:必达标项(2)系统带宽支持10MHz带宽的灵活配置优先级:必达标项(3)子载波间隔15kHz除eMBMS业务外,TD-LTE系统子载波间隔设置为15kHz优先级:必达标项1.2子帧配置(1)帧结构支持上下行时隙配比5ms 2DL:2UL、3DL:1UL,其他配置可软件升级实现优先级:必达标项(2)帧结构支持特殊时隙配比10:2:2,3:9:2,其他配置可软件升级实现优先级:必达标项(3)帧结构支持Normal CP,用于规避多径引起的干扰(Normal CP可规避最大4.6us内的符号间干扰)优先级:必达标项(4)特殊时隙的应用支持在DwPTS上传送物理层控制信道(PDCCH/ PCFICH/PHICH);当DwPTS上符号数不小于6时,支持同时发送数据信道优先级:必达标项(5)特殊时隙的应用支持配置终端在特殊时隙UpPTS上发送Sounding参考信号,并能正确接收优先级:必达标项1.3随机接入(1)随机接入系统支持随机接入PRACH format 0优先级:必达标项(2)随机接入随机接入支持发送密度为10ms 1次和20ms一次优先级:必达标项(3)随机接入支持基于竞争和非竞争的随机接入,并且运营商可配置基于竞争和非竞争随机接入码数量(每个小区可分配的随机接入码数量为64个)优先级:必达标项1.4上行功控(1)上行功控支持运营商配置PRACH功率初始值和功率调整步长(powerRampingStep)优先级:必达标项(2)上行功控支持PRACH 消息3(msg3)的闭环功控,提高接入成功率优先级:必达标项(3)上行功控支持PUSCH、PUCCH、Sounding参考信号闭环功控优先级:必达标项(4)上行功控支持功率余量power headroom的事件触发性和周期性两种反馈触发机制,且支持触发周期和门限的设置优先级:必达标项(5)上行功控支持单个功控命令用于承载多个UE的功率调整信息(DCI format 3)以提高传输效率优先级:必达标项(6)上行功控支持PUSCH根据上行接收信号强度或质量、Power Head Room、接收机性能、MCS解调门限等因素来调整终端的发射功率优先级:必达标项(7)上行功控支持PUSCH绝对式和累积式的功率控制优先级:必达标项1.5空口同步(1)空口同步在启动阶段,皮站通过监听其他基站PSS/SSS获得其他基站的同步信息,并通过侦听宏基站CRS跟踪和保持同步情况。
LTE 射频测试操作指南
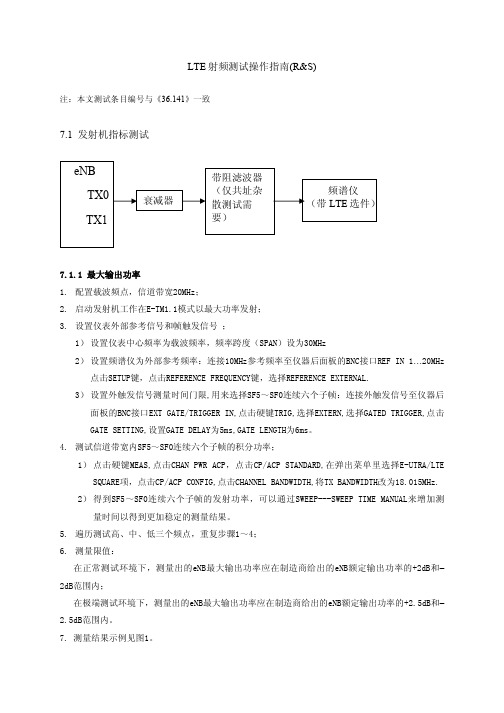
20
20
8. 测量结果示例见图5.
图5 单次测量的OFDM SYMBOL的平均功率
7.1.4 发射关断功率(选项) 1. 配置载波频点,信道带宽20MHz; 2. 启动发射机工作在E-TM1.1模式以最大功率发射 3. 进 入 LTE 测 试 选 件 , 点 击 FILE---LOAD DEMOD SETTING, 在 弹 出 的 菜 单 中 选 择 E-TM 1_1_20MHz.ALLOCATION, 点 击 MEAS---GENERAL SETTING, 在 弹 出 的 菜 单 中 设 置 双 工 方 式 (DUPLEXING):TDD; 链路方向(LINK DIRECTION):DOWNLINK;频率(FREQUENCY):发射机载波 频率;被分析信号来源(INPUT SOURCE):RF;自动幅度电平(AUTO LEVEL):YES;触发设置中触 发模式(TRIGGER MODE)设为:外触发(EXTERNAL);触发延时(TRIGGER OFFSET)设为: 0s。点击MEAS--PVT,然后点击主界面的调整时间(ADJUST TIMING),时间调整完毕后, 点击主界面的单次测量 (RUN SGL),得到测量结果。 4. 测量结果示例见图6。
图6 发射关断功率结果示图 7.1.5 发射开关时间模板(选项) 1. 配置载波频点,信道带宽20MHz; 2. 启动发射机工作在E-TM1.1模式以最大功率发射 3. 进 入 LTE 测 试 选 件 , 点 击 FILE---LOAD DEMOD SETTING, 在 弹 出 的 菜 单 中 选 择 E-TM 1_1_20MHz.ALLOCATION, 点 击 MEAS---GENERAL SETTING, 在 弹 出 的 菜 单 中 设 置 双 工 方 式 (DUPLEXING):TDD; 链路方向(LINK DIRECTION):DOWNLINK;频率(FREQUENCY):发射机载波 频率;被分析信号来源(INPUT SOURCE):RF;自动幅度电平(AUTO LEVEL):YES;触发设置中触 发模式(TRIGGER MODE)设为:外触发(EXTERNAL);触发延时(TRIGGER OFFSET)设为: 0s。点击MEAS-
移动终端中LTE和WIFI共存干扰研究
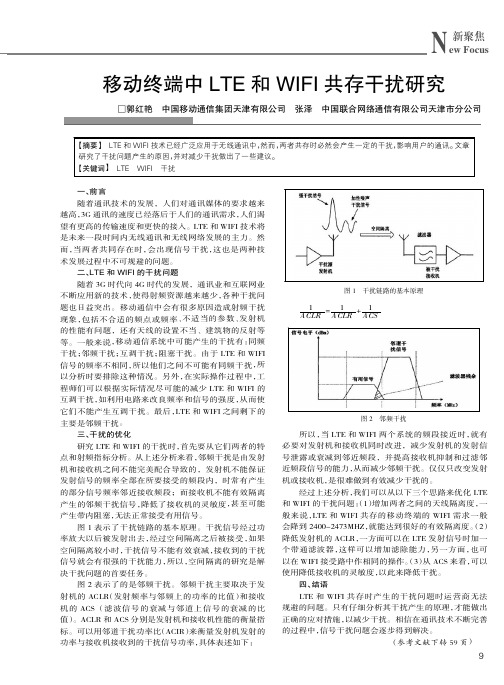
移动终端中LTE 和WIFI 共存干扰研究□郭红艳中国移动通信集团天津有限公司张泽中国联合网络通信有限公司天津市分公司一、前言随着通讯技术的发展,人们对通讯媒体的要求越来越高,3G 通讯的速度已经落后于人们的通讯需求,人们渴望有更高的传输速度和更快的接入。
LTE 和WIFI 技术将是未来一段时间内无线通讯和无线网络发展的主力。
然而,当两者共同存在时,会出现信号干扰,这也是两种技术发展过程中不可规避的问题。
二、LTE 和WIFI 的干扰问题随着3G 时代向4G 时代的发展,通讯业和互联网业不断应用新的技术,使得射频资源越来越少,各种干扰问题也日益突出。
移动通信中会有很多原因造成射频干扰现象,包括不合适的频点或频率、不适当的参数、发射机的性能有问题,还有天线的设置不当、建筑物的反射等等。
一般来说,移动通信系统中可能产生的干扰有:同频干扰;邻频干扰;互调干扰;阻塞干扰。
由于LTE 和WIFI 信号的频率不相同,所以他们之间不可能有同频干扰,所以分析时要排除这种情况。
另外,在实际操作过程中,工程师们可以根据实际情况尽可能的减少LTE 和WIFI 的互调干扰,如利用电路来改良频率和信号的强度,从而使它们不能产生互调干扰。
最后,LTE 和WIFI 之间剩下的主要是邻频干扰。
三、干扰的优化研究LTE 和WIFI 的干扰时,首先要从它们两者的特点和射频指标分析。
从上述分析来看,邻频干扰是由发射机和接收机之间不能完美配合导致的,发射机不能保证发射信号的频率全部在所要接受的频段内,时常有产生的部分信号频率邻近接收频段;而接收机不能有效隔离产生的邻频干扰信号,降低了接收机的灵敏度,甚至可能产生带内阻塞,无法正常接受有用信号。
图1表示了干扰链路的基本原理。
干扰信号经过功率放大以后被发射出去,经过空间隔离之后被接受,如果空间隔离较小时,干扰信号不能有效衰减,接收到的干扰信号就会有很强的干扰能力,所以,空间隔离的研究是解决干扰问题的首要任务。
TD-LTE基站射频测试步骤详解
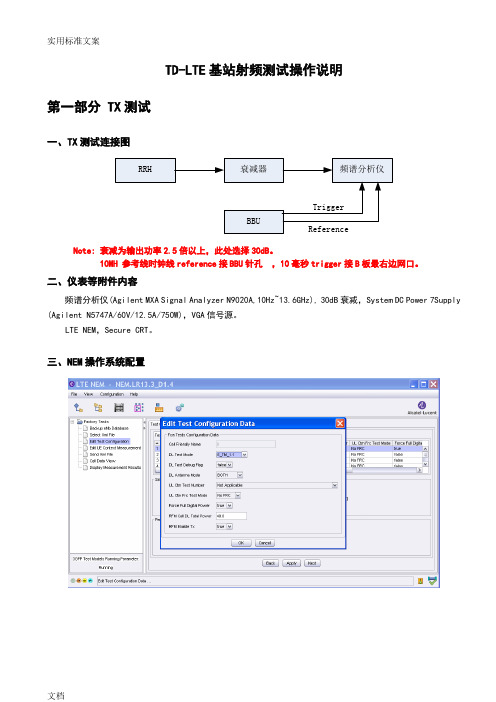
实用标准文案TD-LTE基站射频测试操作说明第一部分 TX测试一、TX测试连接图RRH衰减器频谱分析仪BBUTrigger ReferenceNote: 衰减为输出功率2.5倍以上,此处选择30dB。
10MH 参考线时钟线reference接BBU针孔,10毫秒trigger接B板最右边网口。
二、仪表等附件内容频谱分析仪(Agilent MXA Signal Analyzer N9020A,10Hz~13.6GHz), 30dB衰减,System DC Power 7Supply (Agilent N5747A/60V/12.5A/750W),VGA信号源。
LTE NEM,Secure CRT。
三、NEM操作系统配置四、频谱仪选键设置Step 1. 选择LTE模式,Press Mode, LTE TDD.Step 2. 频点设置FREQ ChannelStep 3. 频点补偿设置Input/Output, External Gain, BTSStep 4. 下行模式设置Mode Setup, Radio, Direction to be Downlink.Step 5. 链路配置设Mode Setup, Radio, ULDLAlloc, Config 3;DW/GP/Up Len, More, Config 8.Step 6. 时间门限设置Sweep/control, Gate, Gate Delay=5ms, Gate Length=6.8msStep 7. 带宽设置Mode setup, Prest To Standard=10/20MHzStep 8. 触发方式设置Trigger, External 1/2配置完后频谱分析仪显示如下:五、下行发射项测试操作步骤1. 输出功率Output Power测试项选择 Meas---Channel power测试模式 E-TM1.1Note: 查看Channel Power值,(10W标准值40dBm)。
LTE系统中ACLR的测量
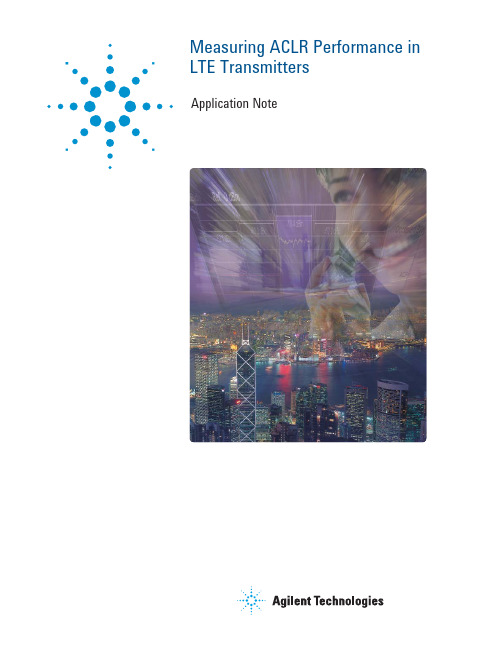
Measuring ACLR Performance in LTE TransmittersApplication NoteIntroductionAs wireless service providers push for more bandwidth to deliver IP services to more users, LTE has emerged as a next-generation cellular technology with great potential to enhance current deployments of 3GPP networks and to enable sig-nificant new service opportunities. However, LTE’s complex, evolved architecture introduces new challenges in designing and testing network and user equipment. The commercial success of LTE will depend in part on the ability of all devices to work as specified. One of the particular challenges at the air interface will be power management during signal transmission. In a digital communication system such as LTE, the power that leaks from a transmitted signal into adjacent channels can interfere with transmissions in the neighboring channels and impair system performance. The adjacent channel leakage-power ratio (ACLR) test verifies that system transmitters are performing within specified limits. This critical yet complex transmitter test can be made quickly and accurately using modern signal analyzers such as the Agilent X-Series (PXA/MXA/EXA) signal analyzers with LTE measurement software and signal generators such as the Agilent MXG signal generator with LTE signal creation software.LTE product development is underway, and RF engineers are tackling the many design and measurement challenges this complex technology presents. LTErequires support for six channel bandwidths (from 1.4 to 20 MHz), different transmission schemes for the downlink and the uplink (OFDMA and SC-FDMA), two transmission modes (FDD and TDD), and multiple antenna techniques (MIMO spatial multiplexing, diversity, beamsteering). As a result of LTE’s flexible transmission schemes, the physical channel configuration has a large impact on RF performance—much greater than in current CDMA-based systems. With per-formance targets set exceptionally high for LTE, engineers have to make careful design tradeoffs to cover each critical part of the radio transmitter chain.One important aspect of transmitter design is the need to minimize unwanted emissions. Because LTE will be deployed in the same frequency bands as W-CDMA and other legacy cellular technologies, the 3GPP specifications regu-late emissions to minimize interference and ensure compatibility between the different radio systems. The primary concern is control of spurious emissions, which can occur at any frequency. In this respect LTE is similar to other radio systems. However, new challenges arise at the band edges, where the transmit-ted signal must comply with rigorous power leakage requirements. With LTE supporting channel bandwidths up to 20 MHz, and with many bands too narrow to support more than a few channels, a large proportion of the LTE channels will be at the edge of the band.Controlling transmitter performance at the edge of the band requires a design with filtering to attenuate out-of-band emissions without affecting in-channel performance. Factors such as cost, power efficiencies, physical size, and location in the transmitter block diagram are also important considerations. Ultimately the LTE transmitter must meet all specified limits for unwanted emissions, including limits on the amount of power that leaks into adjacent channels, as defined by ACLR.Challenges of LTE transmitterdesignACLR test requirements ACLR is a key transmitter characteristic included in the LTE RF transmitterconformance tests (Table 1). These tests verify that minimum requirements arebeing met in the base station (eNB) and user equipment (UE). Most of the LTEconformance tests for out-of-band emissions are similar in scope and purposeto those for W-CDMA and should look familiar. However, while W-CDMA speci-fies a root-raised cosine (RRC) filter for making transmitter measurements, noequivalent filter is defined for LTE. Thus different filter implementations canbe used for LTE transmitter testing to optimize either in-channel performance,resulting in improved error vector magnitude, or out-of-channel performance,resulting in better adjacent channel power characteristics.Table 1. Conformance tests for RF transmitters (from 3GPP TS 36.141 [1] and 36.521-1 [2]) ArrayGiven the extensive number of complex transmitter configurations that canbe used to test transmitter performance, LTE specifies a series of downlinksignal configurations known as E-UTRA test models (E-TM) for testing theeNB. These test models are grouped into three classes: E-TM1, E-TM2, andE-TM3. The first and third classes are further subdivided into E-TM1.1, E-TM1.2,E-TM3.1, E-TM3.2, and E-TM3.3 (Table 2). Note that the “E” in E-UTRA stands for“enhanced” and designates LTE UMTS terrestrial radio access, whereas UTRAwithout the “E” refers to W-CDMA.ACLR test requirements (continued)For UE testing, transmitter tests are carried out using the reference measurement channels (RMC) specified for eNB receiver testing. The ACLR requirement for the UE is not as stringent as it is for the eNB, so our focus will be on the latter. Table 2. E-UTRA test models (from 3GPP TS 36.141 [1])The 3GPP specifications for LTE define ACLR as the ratio of the filtered mean power centered on the assigned channel frequency to the filtered mean power centered on an adjacent channel frequency. Minimum ACLR conformancerequirements for the eNB are given for two scenarios: for adjacent E-UTRA (LTE) channel carriers of the same bandwidth, E-UTRAACLR1, and for the UTRA (W-CDMA) adjacent and alternate channel carriers, UTRAACLR1and UTRAACLR2, respectively.Different limits and measurement filters are specified for E-UTRA and UTRA adja-cent channels, and are provided for both paired spectrum (FDD) operation and unpaired spectrum (TDD) operation. The E-UTRA channels are measured using a square measurement filter, while UTRA channels are measured using an RRC filter with a roll-off factor of = 0.22 and a bandwidth equal to the chip rate, which is 3.84 MHz in the example of paired spectrum operation shown in Figure 1.E-TM1.1Maximum power testsOutput power, occupied bandwidth, ACLR,operating band unwanted emissions,transmitter spurious emissions, transmitterintermodulation, reference signal absoluteaccuracyE-TM1.2Includes power boosting andde-boostingACLR, operating band unwanted emissionsE-TM2Minimum power testsTotal power dynamic range (lower OFDMsymbol power limit at min power, EVMof single 64QAM PRB allocation (at minpower), frequency error (at min power)E-TM3.1Power testsTotal power dynamic range (upper OFDMsymbol power limit at max power with all64QAM PRBs allocated), frequency error,EVM for 64QAM (at max power)E-TM3.2Includes power boosting andde-boostingFrequency error, EVM for 16QAME-TM3.3Includes power boosting andde-boostingFrequency error, EVM for QPSKFigure 1. Measurement filters for ACLRmeasurementsACLR test requirements (continued)ACLR test requirements for the eNB including paired and unpaired spectrum operation are summarized in Table 3. As of the September 2009 specification release, the ACLR test cases for the UE were not fully complete. However, the UE test procedure is essentially the same as that used for the base station. Table 3. ACLR base station conformance test requirements (from 3GPP TS 36.141 6.2 [1]). Note that the specification defines the minimum requirement plus the test tolerance (TT)).* Relative limits are 44.2 dB = 45 dB min requirement + 0.8 dB TT. Both Absolute and Relative limits are provided. Whichever is less stringent is to be used for the conformance requirement. Sophisticated signal evaluation tools are available for making complex LTE measure-ments quickly and accurately. Power measurements including ACLR generally are made using a spectrum or signal analyzer, and the required test signals are built using a signal generator. In the following examples, Agilent’s PC-based Signal Studio application connected to an MXG signal generator is used to build the standards-compliant E-TM signal required for transmitter testing. The output signal is connected to the RF input of an Agilent X-Series signal analyzer running N9080A LTE measure-ment application, which is used for signal analysis. This equipment setup follows the simple block diagram provided in the 3GPP LTE specifications (Figure 2). Although the measurement process described here is for FDD operation, the process for TDD operation is similar.According to the specifications, the carrier frequency must be set within a frequency band supported by the base station under test, and ACLR must be measured for frequency offsets on both sides of the channel frequency, as specified for paired or unpaired spectrum operation (T able 3). The test is performed first using a transmitted signal of type E-TM1.1, in which all of the PDSCH resource blocks have the same power, and then again using E-TM1.2, in which power boosting and deboosting are used. The E-TM1.2 configuration is useful because it simulates multiple users whose devices are operating at different power levels. This scenario results in a higher crest factor, which makes it more difficult to amplify the signal without creating additional, unwanted spectral content—i.e., ACLR.PairedSpectrumCategory AE-UTRA 44.2 dB or -13 dBm/MHzUTRA 44.2 dB or -13 dBm/MHz PairedSpectrumCategory BE-UTRA 44.2 dB or -15 dBm/MHzUTRA 44.2 dB or -15 dBm/MHzUnpairedspectrumCategory AE-UTRA (LTE) 44.2 dB or -13 dBm/MHz1.28 Mcps UTRA 44.2 dB or -13 dBm/MHz3.84 Mcps UTRA 44.2 dB or -13 dBm/MHz7.82 Mcps UTRA 44.2 dB or -13 dBm/MHzUnpairedspectrumCategory BE-UTRA (LTE) 44.2 dB or -15 dBm/MHz1.28 Mcps UTRA 44.2 dB or -15 dBm/MHz3.84 Mcps UTRA 44.2 dB or -15 dBm/MHz7.82 Mcps UTRA 44.2 dB or -15 dBm/MHzSetting up the ACLR testFigure 2. Measurement equipment setup (3GPP TS 36.141 [1] Annex I, Figure I.1-1)Setting up the ACLR test (continued)In this ACLR measurement example, Signal Studio is set up to generate a standards-compliant E-TM1.2 test signal. The frequency is set to 2.11 GHz, a frequency that is in several of the major downlink operating bands specified for LTE. The output signal amplitude—an important consideration in determining ACLR performance—is set to -10 dBm. A 5 MHz channel bandwidth is selected from the range that extends from 1.4 to 20 MHz.Figure 3 shows the eNB setup with Transport Channel selected. A graph of the resource allocation blocks for the test signal appears at the bottom. The Y axis indicates frequency or resource blocks, while the X axis indicates slots or time. The different colors correspond to different channels, with the white areas representing Channel 1 and the pink areas Channel 2. Both are downlink shared channels, of interest in this measurement. The other colors represent synchroni-zation channels, reference signals, etc.Figure 3. Resource allocation blocks (at bottom) for E-TM1.2 test signalSelecting Channel 1 shows the output power level to be at -4.3 dB, so the channel power has been deboosted. The output power of Channel 2 has been boosted and is set at 3 dB (Figure 4). A complex array of power boosting and deboosting options can be set for the different resource blocks from resource block allocation graph. The resulting composite signal will have a higher peak-to-average ratio than a single channel in which all blocks are at the same power level. Amplifying a boosted signal such as this can be difficult, as noted earlier. Without sufficient back-off in the power amplifier, clipping may result.Figure 4. Boosted output power in Channel 2Setting up the ACLR test (continued)The test signal is now generated using the Signal Studio software. Because Signal Studio is PC-based, it can be run from the PC-based X-Series signal ana-lyzer. The waveform in this case is created on the desktop of the signal analyzer and then downloaded to the signal generator via LAN or GPIB. The RF output of the signal generator is connected to the RF input of the signal analyzer, where the ACLR performance is measured using swept spectrum analysis. In this example, the signal analyzer is in LTE mode with a center frequency of 2.11 GHz and the ACP measurement selected. At this point it is possible to make a quick, one-button ACLR measurement according to the LTE standard by recalling the appropriate parameters and test limits from a list of available choices in the LTE application. These choices include options for paired and unpaired spectrum, Category A or Category B limits (as defined in ITU-R SM.329), and type of carrier in the adjacent and alternate channels—E-UTRA (LTE), UTRA (W-CDMA), or TD-SCDMA.Recall that for FDD operation, LTE defines two different methods of making ACLR measurements. In Figure 5, the upper graph shows the case in which E-UTRA (LTE) is used at the center and offset frequencies. The lower graph shows LTE at the center frequency and UTRA (W-CDMA) at the adjacent and alternate offsets.Figure 5. Two specified methods of ACLR measurementIn Figure 6 the measurement result shows the E-UTRA adjacent and alternate offset channels. For this measurement a 5 MHz carrier is selected; however, the measure-ment noise bandwidth is 4.515 MHz, because the downlink contains 301 subcarriers. The first offset (A) is at 5 MHz, with an integration bandwidth of 4.515 MHz. The second offset (B) is at 10 MHz with the same integration bandwidth.Figure 6. ACLR measurement result using Agilent X-Series analyzer before optimization This one-button measurement gives a very quick, usable ACLR measurementaccording to the LTE standard. While the result in Figure 6 of about -62 dBc is good, the analyzer settings can be optimized to get even better performance. Four ways to further improve the measurement results are (1) optimize the signal level at the mixer; (2) change the resolution bandwidth filter; (3) turn on noise correction; and (4) use a different measurement methodology called filtered integration bandwidth.To optimize the signal level at the input mixer, the attenuator is adjusted for minimal clipping. The X-Series signal analyzer will automatically select an attenuation value based on the current measured signal value. This automated technique provides a good starting point for achieving optimal measurement range. Signal analyzers such as the X-Series, which have both electronic and mechanical attenuators, can use the two in combination to optimize performance. In such cases the mechanical attenuator can be adjusted slightly to get even better results of about 1 or 2 dB.Next the resolution bandwidth can be lowered by pressing the bandwidth filter key. Note that sweep time increases as the resolution bandwidth is lowered. For example, with the MXA signal analyzer, sweep time at 30 kHz is 676.3 ms. At a lower 10 kHz RBW, the sweep time is about 6 seconds. The slower sweep time reduces variance in the measurement, but reduces measurement speed.Another step is to turn on noise correction. The analyzer then takes one sweep to measure its internal noise floor at the current center frequency, and in subse-quent sweeps subtracts that internal noise floor from the measurement result. This technique substantially improves ACLR, in some cases by up to 5 dB.Setting up the ACLR test(continued)Optimizing the analyzer settingsOptimizing the analyzer settings (continued)Changing the measurement method is a fourth way to optimize the analyzer. In this case the default measurement method (integration bandwidth or IBW) is changed to the filtered IBW method. Filtered IBW uses a sharp, steep cutoff filter. This technique does degrade the absolute accuracy of the power measurement result, but it does not degrade the relative power accuracy, and ACLR is a relative power measurement. Therefore, filtered IBW does not degrade the ACLR result. Using these techniques in combination, an Agilent’s X-series analyzer can optimize the ACLR measurement automatically for performance versus speed via the analyzer’s embedded LTE application. The result for a typical ACLR mea-surement is improved by up to 10 dB or more. Figure 7 shows an 11 dB ACLR improvement after optimization (compared to Figure 6) using the embedded LTE application. For measurement scenarios requiring the maximum performance, the analyzer settings can be further adjusted.Figure 7. ACLR measurement result using Agilent X-series analyzer after optimization Standards-compliant spectrum measurements such as ACLR are invaluable for RF engineers developing the next generation radio systems. With LTE, however, these measurements are complicated by factors such as variations in the band-width of adjacent channels, choice of transmission filter, and interaction of RF variables between channels of different bandwidth and different susceptibility to interference. A practical solution is to use a spectrum or signal analyzer with a standards-specific measurement application. This combination can reduce error in complex measurements, automatically configuring limit tables and specifiedtest setups and ensuring measurement repeatability.ConclusionAcronyms3GPP 3rd Generation Partnership ProjectACLR Adjacent channel leakage-power ratioA-MPR Additional maximum power reductionCDMA Code division multiple accesseNB Evolved node BE-TM E-UTRA test modelE-UTRA Evolved universal terrestrial radio accessEVM Error vector magnitudeFDD Frequency division duplexGPIB General purpose interface busI BW I ntegrationbandwidthLAN Local area networkLTE Long term evolutionMPR Maximum power reductionMIMO Multiple input multiple outputOFDMA Orthogonal frequency division multiple accessPRACH Physical random access channelQAM Quadrature amplitude modulationQPSK Quadrature phase-shift keyingblockRB ResourcebandwidthRBW ResolutionelementRE ResourcefrequencyRF RadiocosineRRC Root-raisedSC-FDMA Single carrier frequency division multiple accessSRS Sounding reference signalTDD Time division duplexTD-SCDMA Time domain synchronous code division multiple accessequipmentUE UserUMTS Universal mobile telecommunications systemUTRA Universal terrestrial radio accessW-CDMA Wideband code division multiple accessReferences More Information [1] 3GPP TS 36.141 V8.4.0 (2009-09) Base Station (BS) Conformance Testing[2] 3GPP TS 36.521-1 V8.3.1 (2009-09) User Equipment (UE) ConformanceSpecification; Radio Transmission and Reception Part 1: Conformance T estingFor more information about the 3GPP, visit the 3GPP home page/3GPP specifications home page/specs/specs.htm3GPP Series 36 (LTE) specifications/ftp/Specs/archive/36_seriesFor more information about Agilent design and test products for LTE visit/find/lteAgilent LTE application notes and technical overviews:3GPP Long T erm Evolution: System Overview, Product Development, and Test Challenges: 5989-8139ENLTE Component T est: 5990-5149ENMIMO in LTE Operation and Measurement—Excerpts on LTE T est: 5990-4760EN MIMO Performance and Condition Number in LTE T est: 5990-4759ENN9080A & N9082A LTE Modulation Analysis Measurement Application T echnical Overview: 5989-6537ENLearn more about LTE and its measurements in the new book written by 30 LTE experts:LTE and the Evolution to 4G Wireless Designand Measurement Challenges/find/ltebook11Remove all doubtOur repair and calibration services will get your equipment back to you, performing like new, when promised. You will get full value out of your Agilent equipment through-out its lifetime. Your equipment will be serviced by Agilent-trained technicians using the latest factory calibration procedures, automated repair diagnostics and genuine parts. You will always have the utmost confidence in your measurements. For information regarding self maintenance of this product, please contact your Agilent office.Agilent offers a wide range of additional expert test and measurement services for your equipment, including initial start-up assistance, onsite education and training, as well as design, system integration, and project management. For more information on repair and calibration services, go to:/find/removealldoubtAgilent Email Updates/find/emailupdates Get the latest information on the products and applications you select.LXI is the LAN-based successor to GPIB, providing faster, more efficient connectivity. Agilent is a founding member of the LXI consortium.For more information on Agilent Technologies’ products, applications or services, please contact your local Agilent office. The complete list is available at:/find/contactusAmericasCanada (877) 894-4414 Latin America 305 269 7500United States (800) 829-4444Asia Pacific Australia 1 800 629 485China800 810 0189Hong Kong 800 938 693India 1 800 112 929Japan 0120 (421) 345Korea 080 769 0800Malaysia 1 800 888 848Singapore 1 800 375 8100Taiwan 0800 047 866Thailand1 800 226 008Europe & Middle East Austria 43 (0) 1 360 277 1571Belgium 32 (0) 2 404 93 40 Denmark 45 70 13 15 15Finland 358 (0) 10 855 2100France 0825 010 700**0.125 €/minuteGermany 49 (0) 7031 464 6333 Ireland 1890 924 204Israel 972-3-9288-504/544Italy 39 02 92 60 8484Netherlands 31 (0) 20 547 2111Spain 34 (91) 631 3300Sweden 0200-88 22 55Switzerland 0800 80 53 53United Kingdom 44 (0) 118 9276201Other European Countries:/find/contactusRevised: October 1, 2009Product specifications and descriptions in this document subject to change without notice.© Agilent Technologies, Inc. 2009, 2010Printed in USA, January 7, 20105990-5089EN/find/lteAgilent Channel Partnersw w w /find/channelpartners Get the best of both worlds: Agilent’s measurement expertise and product breadth, combined with channel partner convenience.。
LTE发射机ACLR性能的测量技术

LTE发射机ACLR性能测量技术作者: Jung-ik Suh安捷伦科技企业上网日期: 12月17日已经有[ 0 ]个评论打印版订阅关键字: LTE发射机ACLR性能LTE测量发射滤波器现代无线服务提供商正致力于不停扩大带宽, 为更多用户提供互联网协议(IP)服务。
长久演进技术(LTE)是新一代蜂窝技术, 能够增强目前布署3GPP网络并发明关键新业务机会, 从而满足上述需求。
LTE体系结构复杂而且还在不停演进, 这为网络和用户设备设计与测试带来了新挑战。
其中, 在空中接口上一个关键挑战就是怎样在信号传输过程中进行功率管理。
在LTE等数字通信系统中, 发射信号泄漏到邻近信道功率可能会对邻近信道中信号传输产生干扰, 进而影响系统性能。
相邻信道泄漏功率比(ACLR)测试能够验证系统发射机工作性能是否符合要求限制。
鉴于LTE技术复杂性, 快速和正确地实施这种关键测试对于测试人员来说可能充满挑战性(见表1)。
装有LTE特定信号生成软件信号发生器、装有LTE特定测量软件现代化信号分析仪, 以及针对该分析仪优化方法, 能够帮助测试人员战胜这一挑战。
了解ACLR测试要求ACLR是LTE射频发射机一致性测试中一个关键发射机特征。
这些测试目是验证被测件是否达成了基站(eNB)和用户设备(UE)中最低要求。
大部分针对带外发射LTE一致性测试与在范围和目上与针对WCDMA一致性测试类似。
不过WCDMA指定了使用根余弦(RRC)滤波器进行发射机测量, 而标准并没有为LTE定义等效滤波器。
所以, LTE发射机测试能够使用不一样滤波器来优化信道带内性能, 改善误差矢量幅度; 或优化信道带外性能, 取得更出色邻近信道功率特征。
鉴于在测试发射机性能中能够使用复杂发射机配置有很多, LTE指定了一系列下行链路信号配置来测试eNB。
这些配置称为E-UTRA测试模型(E-TM)。
它们可分为三大类: E-TM1、E-TM2和E-TM3。
LTE基站发射机本振信号的主要指标与测试方法
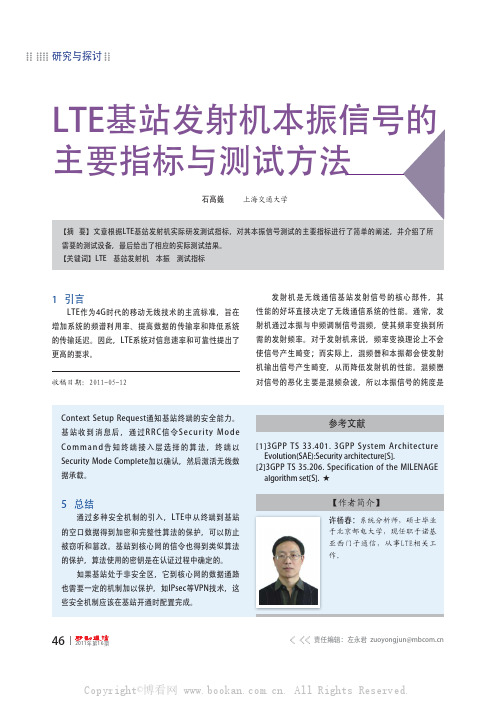
研究与探讨责任编辑:左永君*******************462011年第16期【摘 要】文章根据LTE基站发射机实际研发测试指标,对其本振信号测试的主要指标进行了简单的阐述,并介绍了所需要的测试设备,最后给出了相应的实际测试结果。
【关键词】LTE 基站发射机 本振 测试指标收稿日期:2011-05-12石高巍 上海交通大学LTE基站发射机本振信号的1 引言LTE作为4G时代的移动无线技术的主流标准,旨在增加系统的频谱利用率、提高数据的传输率和降低系统的传输延迟。
因此,LTE系统对信息速率和可靠性提出了更高的要求。
发射机是无线通信基站发射信号的核心部件,其性能的好坏直接决定了无线通信系统的性能。
通常,发射机通过本振与中频调制信号混频,使其频率变换到所需的发射频率。
对于发射机来说,频率变换理论上不会使信号产生畸变;而实际上,混频器和本振都会使发射机输出信号产生畸变,从而降低发射机的性能。
混频器对信号的恶化主要是混频杂波,所以本振信号的纯度是Context Setup Request通知基站终端的安全能力。
基站收到消息后,通过R R C 信令S e c u r i t y M o d e C o m m a n d 告知终端接入层选择的算法,终端以Security Mode Complete加以确认,然后激活无线数据承载。
5 总结通过多种安全机制的引入,LTE中从终端到基站的空口数据得到加密和完整性算法的保护,可以防止被窃听和篡改。
基站到核心网的信令也得到类似算法的保护,算法使用的密钥是在认证过程中确定的。
如果基站处于非安全区,它到核心网的数据通路也需要一定的机制加以保护,如IPsec等VPN技术,这些安全机制应该在基站开通时配置完成。
参考文献[1]3GPP TS 33.401. 3GPP System Architecture Evolution(SAE):Security architecture[S].[2]3GPP TS 35.206. Specification of the MILENAGE algorithm set[S]. ★【作者简介】许杨春:系统分析师,硕士毕业于北京邮电大学,现任职于诺基亚西门子通信,从事LTE相关工作。
关于3GPP标准中基站频谱发射模板和ACLR两个指标的考虑
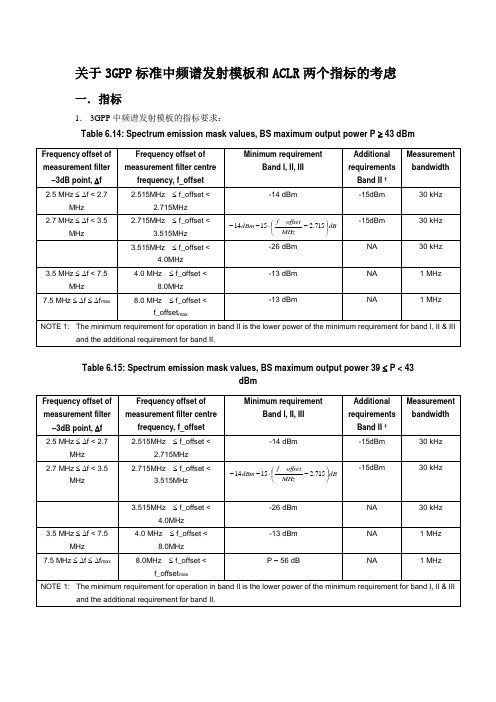
关于3GPP标准中频谱发射模板和ACLR两个指标的考虑一.指标1.3GPP中频谱发射模板的指标要求:Table 6.14: Spectrum emission mask values, BS maximum output power P ≥ 43 dBmTable 6.15: Spectrum emission mask values, BS maximum output power 39 ≤ P < 43dBmTable 6.16: Spectrum emission mask values, BS maximum output power 31 ≤ P < 39dBmTable 6.17: Spectrum emission mask values, BS maximum output powerP < 31 dBm2. 3GPP 中ACLR 的指标要求:Table 6.22: BS ACLR二.问题的提出:在WCDMA高功放的测试中发现,在单载波满足ACLR指标要求时,频谱发射模板要求并不满足,必须将输出功率回退,使其临道ACLR指标达到-48dBc左右,才有可能能满足频谱发射模板要求。
为什么同为临近频带的线性指标要求,ACLR能满足指标甚至留有余量2dB左右,而频谱发射模板指标却过不去?三.分析●定义分析:1.共性:频谱发射模板和ACLR两个指标在3GPP中是同属于“带外发射(out of bandemission)”指标。
带外发射的定义是:由调制过程和传输中的非线性产生的紧邻有用信道外的有害发射,不包括杂散发射。
2.区别:A. 适用范围不同。
频谱发射模板只是在特定的一些区域需要满足的一个指标,而在其他某些地域则不一定要求。
ACLR指标则是在任何情况都必须满足。
ACLR指标只是针对WCDMA系统自身干扰而言的,也就是不希望对同一系统内工作在其相邻载波的其他基站造成干扰。
TD—LTE系统干扰分析
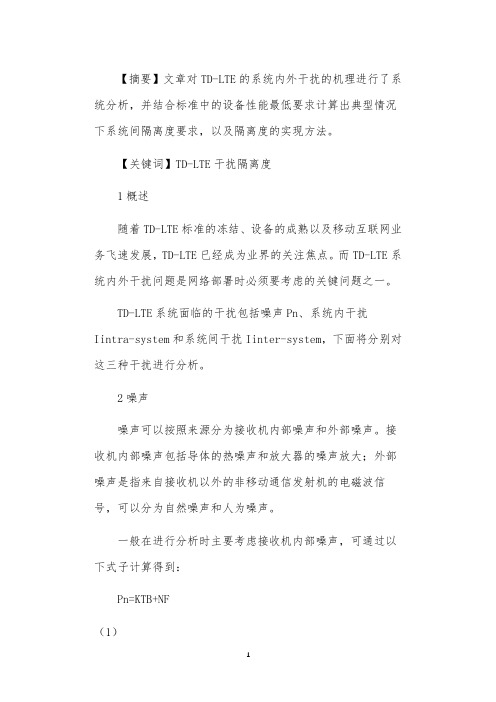
【摘要】文章对TD-LTE的系统内外干扰的机理进行了系统分析,并结合标准中的设备性能最低要求计算出典型情况下系统间隔离度要求,以及隔离度的实现方法。
【关键词】TD-LTE干扰隔离度1概述随着TD-LTE标准的冻结、设备的成熟以及移动互联网业务飞速发展,TD-LTE已经成为业界的关注焦点。
而TD-LTE系统内外干扰问题是网络部署时必须要考虑的关键问题之一。
TD-LTE系统面临的干扰包括噪声Pn、系统内干扰Iintra-system和系统间干扰Iinter-system,下面将分别对这三种干扰进行分析。
2噪声噪声可以按照来源分为接收机内部噪声和外部噪声。
接收机内部噪声包括导体的热噪声和放大器的噪声放大;外部噪声是指来自接收机以外的非移动通信发射机的电磁波信号,可以分为自然噪声和人为噪声。
一般在进行分析时主要考虑接收机内部噪声,可通过以下式子计算得到:Pn=KTB+NF(1)其中:K:波尔兹曼常数(Boltzmannconstant),1.380662×10-23JK-1;T:开尔文绝对温度,一般计算中取常温290K;B:接收机有效带宽;NF:接收机的噪声系数,标准中一般取基站的噪声系数分别为7dB。
由于LTE系统带宽在1.4MHz~20MHz可变,并且采用OFDMA/SC-FDMA的多址方式,用户实际只占用系统带宽中的一部分。
因此,信道的热噪声水平也会随着占用带宽的变化而变化。
3系统内干扰系统内干扰是本移动通信系统内各无线网元收发单元之间的干扰。
3.1同频干扰TD-LTE系统同小区下的不同用户下行采用OFDMA、上行采用SC-FDMA的多址方式,不同用户占用不同的、相互正交的子载波,因此不存在3G系统中的同小区不同用户的多址干扰问题。
LTE系统中的同频干扰主要是同频的其他小区的干扰,这也是LTE系统中干扰协调、抑制技术要解决的问题。
3.2LTETDD系统上下行链路间干扰LTETDD系统采用时分双工的方式,上下行信道工作在相同的频点,通过上下行转换点设置上下行信道可占用的时隙。
LTE基本项的测试
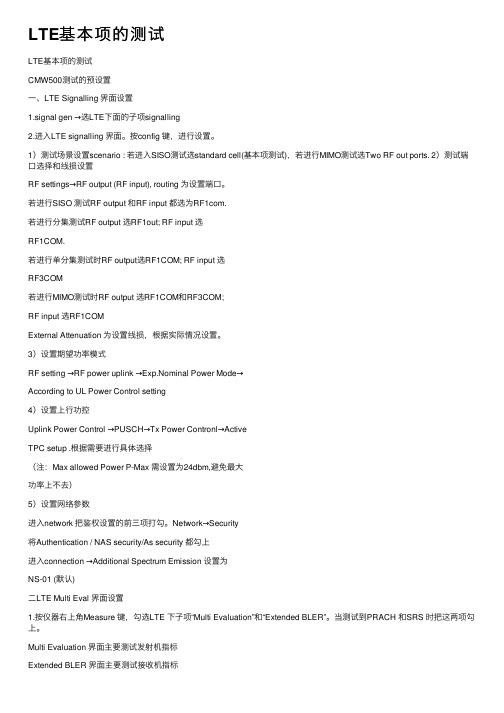
LTE基本项的测试LTE基本项的测试CMW500测试的预设置⼀、LTE Signalling 界⾯设置1.signal gen →选LTE下⾯的⼦项signalling2.进⼊LTE signalling 界⾯。
按config 键,进⾏设置。
1)测试场景设置scenario : 若进⼊SISO测试选standard cell(基本项测试),若进⾏MIMO测试选Two RF out ports. 2)测试端⼝选择和线损设置RF settings→RF output (RF input), routing 为设置端⼝。
若进⾏SISO 测试RF output 和RF input 都选为RF1com.若进⾏分集测试RF output 选RF1out; RF input 选RF1COM.若进⾏单分集测试时RF output选RF1COM; RF input 选RF3COM若进⾏MIMO测试时RF output 选RF1COM和RF3COM;RF input 选RF1COMExternal Attenuation 为设置线损,根据实际情况设置。
3)设置期望功率模式RF setting →RF power uplink →Exp.Nominal Power Mode→According to UL Power Control setting4)设置上⾏功控Uplink Power Control →PUSCH→Tx Power Contronl→ActiveTPC setup .根据需要进⾏具体选择(注:Max allowed Power P-Max 需设置为24dbm,避免最⼤功率上不去)5)设置⽹络参数进⼊network 把鉴权设置的前三项打勾。
Network→Security将Authentication / NAS security/As security 都勾上进⼊connection →Additional Spectrum Emission 设置为NS-01 (默认)⼆LTE Multi Eval 界⾯设置1.按仪器右上⾓Measure 键,勾选LTE 下⼦项“Multi Evaluation”和“Extended BLER”。
基于5G宽带通信信号的ACLR测试方法设计与实现
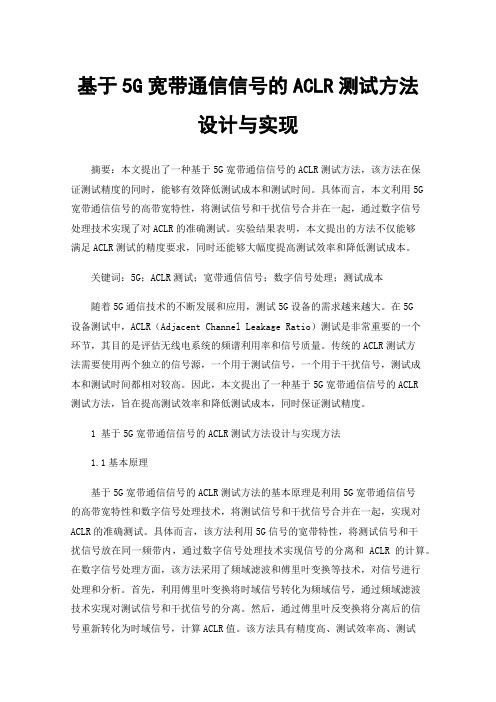
基于5G宽带通信信号的ACLR测试方法设计与实现摘要:本文提出了一种基于5G宽带通信信号的ACLR测试方法,该方法在保证测试精度的同时,能够有效降低测试成本和测试时间。
具体而言,本文利用5G宽带通信信号的高带宽特性,将测试信号和干扰信号合并在一起,通过数字信号处理技术实现了对ACLR的准确测试。
实验结果表明,本文提出的方法不仅能够满足ACLR测试的精度要求,同时还能够大幅度提高测试效率和降低测试成本。
关键词:5G;ACLR测试;宽带通信信号;数字信号处理;测试成本随着5G通信技术的不断发展和应用,测试5G设备的需求越来越大。
在5G设备测试中,ACLR(Adjacent Channel Leakage Ratio)测试是非常重要的一个环节,其目的是评估无线电系统的频谱利用率和信号质量。
传统的ACLR测试方法需要使用两个独立的信号源,一个用于测试信号,一个用于干扰信号,测试成本和测试时间都相对较高。
因此,本文提出了一种基于5G宽带通信信号的ACLR测试方法,旨在提高测试效率和降低测试成本,同时保证测试精度。
1 基于5G宽带通信信号的ACLR测试方法设计与实现方法1.1基本原理基于5G宽带通信信号的ACLR测试方法的基本原理是利用5G宽带通信信号的高带宽特性和数字信号处理技术,将测试信号和干扰信号合并在一起,实现对ACLR的准确测试。
具体而言,该方法利用5G信号的宽带特性,将测试信号和干扰信号放在同一频带内,通过数字信号处理技术实现信号的分离和ACLR的计算。
在数字信号处理方面,该方法采用了频域滤波和傅里叶变换等技术,对信号进行处理和分析。
首先,利用傅里叶变换将时域信号转化为频域信号,通过频域滤波技术实现对测试信号和干扰信号的分离。
然后,通过傅里叶反变换将分离后的信号重新转化为时域信号,计算ACLR值。
该方法具有精度高、测试效率高、测试成本低等优点,能够满足5G设备测试的需求。
需要注意的是,该方法需要对测试信号和干扰信号进行合理的设计和调试,确保信号间的相互独立和合理分布,以避免测试误差和干扰。
- 1、下载文档前请自行甄别文档内容的完整性,平台不提供额外的编辑、内容补充、找答案等附加服务。
- 2、"仅部分预览"的文档,不可在线预览部分如存在完整性等问题,可反馈申请退款(可完整预览的文档不适用该条件!)。
- 3、如文档侵犯您的权益,请联系客服反馈,我们会尽快为您处理(人工客服工作时间:9:00-18:30)。
LTE发射机ACLR性能的测量技术
作者: Jung-ik Suh安捷伦科技公司上网日期: 2012年12月17日已有[0 ]个评论打印版订阅
关键字:LTE发射机 ACLR性能 LTE测量发射滤波器
现代无线服务提供商正致力于不断扩大带宽,为更多用户提供互联网协议(IP)服务。
长期演进技术(LTE)是新一代蜂窝技术,能够增强当前部署的3GPP网络并创造重要的新业务机会,从而满足上述需求。
LTE的体系结构复杂而且还在不断演进,这为网络和用户设备的设计与测试带来了新的挑战。
其中,在空中接口上的一个关键挑战就是如何在信号传输过程中进行功率管理。
在LTE等数字通信系统中,发射信号泄漏到邻近信道的功率可能会对邻近信道中的信号传输产生干扰,进而影响系统性能。
相邻信道泄漏功率比(ACLR)测试可以验证系统发射机的工作性能是否符合规定的限制。
鉴于LTE技术的复杂性,快速和精确地执行这种关键测试对于测试人员来说可能充满挑战性(见表1)。
装有LTE特定信号生成软件的信号发生器、装有LTE特定测量软件的现代化信号分析仪,以及针对该分析仪优化的方法,可以帮助测试人员战胜这一挑战。
了解ACLR测试要求
ACLR是LTE射频发射机一致性测试中的一个重要的发射机特性。
这些测试的目的是验证被测件是否达到了基站(eNB)和用户设备(UE)中的最低要求。
大部分针对带外发射的LTE一致性测试与在范围和目的上与针对WCDMA的一致性测试类似。
但是WCDMA指定了使用根余弦(RRC)滤波器进行发射机测量,而标准并没有为LTE定义等效的滤波器。
因此,LTE发射机测试可以使用不同的滤波器来优化信道带内性能,改善误差矢量幅度;或优化信道带外性能,获得更出色的邻近信道功率特性。
鉴于在测试发射机性能中可以使用的复杂发射机配置有很多,LTE指定了一系列下行链路信号配置来测试eNB。
这些配置称为E-UTRA测试模型(E-TM)。
它们可分为三大类:E-TM1、E-TM2和E-TM3。
第一类和第三类可再细分为E-TM1.1、E-TM1.2、E-TM3.1、E-TM3.2和E-TM3.3。
注:E-UTRA中的“E”源自“enhanced(增强型)”,指LTE UMTS陆地无线接入;而单独的UTRA是指WCDMA。
ACLR测试要求会有所不同,这取决于发射机测试是在UE还是在eNB上进行。
在UE上进行的ACLR测试不像在eNB 上进行那样要求严格。
发射机测试使用规定用于eNB接收机测试的参考测量信道(RMC)来执行。
3GPP LTE规范关于ACLR的定义是,以指定信道频率为中心的滤波后平均功率与以邻近信道频率为中心的滤波后平均功率之比。
eNB的最低ACLR一致性要求分为两种情景指定:相同带宽的邻近E-UTRA信道载波(E-UTRAACLR1);UTRA邻近和相间信道载波(分别是UTRAACLR1和UTRAACLR2)。
针对E-UTRA和UTRA邻近信道规定了不同的限制和测量滤波器,用于成对频谱(FDD)和非成对频谱(TDD)工作。
E-UTRA信道使用平方测量滤波器进行测量,而UTRA信道使用滚降因子为0.22、带宽等于码片速率的RRC滤波器进行测量。
战胜ACLR测量挑战
鉴于LTE技术的复杂性和用于测试发射机性能的发射机配置复杂性,符合标准的频谱测量(例如ACLR)可能非常繁琐。
幸运的是,高级信号测评工具的出现使工程师们能够快速、精确地进行这些LTE测量。
功率测量(包括ACLR)通常使用频谱分析仪或信号分析仪来进行,而要求的测试信号使用信号发生器生成。
为了更好地说明如何使用这些仪器,请设想以下情景:根据规范,载波频率必须设置在被测基站所支持的频段内,按照成对频谱FDD工作或非成对频谱TDD工作时的规定,通过测量信道频率两侧的频偏的ACLR。
首先使用E-TM1.1发射信号进行测试,其中所有PDSCH资源块都具有相同的功率。
然后使用E-TM1.2信号(增加和减少功率)进行测试。
E-TM1.2配置非常有用,因为它能够仿真多个用户(其设备工作在不同功率上)。
这一情景的结果是波峰因数更高,导致在不产生额外无效频谱内容(例如ACLR)的情况下放大信号变得更加困难。
本例中,Agilent Signal Studio for LTE与Agilent MXG信号发生器相连,生成频率设为 2.11GHz且符合标准的E-TM1.2测试信号。
输出信号幅度――决定ACLR性能的重要考虑因素――设为-10dBm。
在从1.4扩展到20MHz 的带宽范围内选择5MHz信道带宽。
图1为已选定传输信道(Transport Channel)的eNB设置。
底部为测试信号的资源分配块图。
信道1和2是要进行测量的信道,它们共享下行链路。
图1:此处显示了E-TM1.2测试信号的资源分配块(底部)。
Y轴表示频率或资源块,X轴表示时隙或时间,白色区域表示信道1,粉红色区域表示信道2,其它颜色表示同步信道、参考信号等。
信道1的输出功率电平为-4.3dB。
因此,其信道功率已经进行过降低。
信道2的输出功率已经进行过增加,设置为3dB。
对于资源块分配图中的不同资源块,可以设置复杂的功率增加和降低选项。
与所有资源块都处于同一功率级的单个信道相比,得到的复合信号具有更高的峰均比。
放大此类功率增加的信号可能非常困难。
功率放大器中没有足够的功率回退(back-off),可能导致限幅。
随后,可以使用在Agilent X系列信号分析仪上运行的Signal Studio软件生成测试信号。
生成信号之后,通过LAN或GPIB将波形下载到信号发生器。
将信号发生器的射频输出端连接到信号分析仪的射频输入端,使用扫描频谱分析测量ACLR性能。
在此例中,信号分析仪处于LTE模式,中心频率为2.11GHz,选择了ACP测量。
随后,通过从LTE应用程序中的一系列可用选项中(例如成对或非成对频谱、邻近信道和相间信道中的载波类型等选项),调用适当的参数和测试限制,根据LTE标准进行快速一键式ACLR测量。
对于FDD测量,LTE定义了两种ACLR测量方法:一种是在中心频率和偏置频率上使用E-UTRA(LTE);另一种是在中心频率上使用LTE,在邻近和相间的偏置频率上使用UTRA(WCDMA)。
图2显示了E-UTRA邻近和相间频偏信道的ACLR测量结果。
对于此次测量,选择5MHz载波,由于下行链路有301个子载波,所以测量噪声带宽为4.515MHz。
图2:此处显示的是使用安捷伦X系列分析仪获得的ACLR测量结果。
第一个频偏(A)位于5MHz处,集成带宽为4.515MHz。
另一个频偏(B)位于10MHz处,具有相同的集成带宽。
化分析仪设置
虽然上述的一键式测量提供了非常快速、易用、依据LTE标准的ACLR测量,但是工程师仍然可以对信号分析仪设置进行优化,获得更出色的性能。
有四种方法可以优化分析仪,进一步改善测量结果:
* 优化混频器上的信号电平――优化输入混频器上的信号电平要求对衰减器进行调整,实现最小的限幅。
有些分析仪能够根据当前测得的信号值自动选择衰减值。
这为实现最佳的测量范围奠定了良好的基础。
其它分析仪(例如X系列信号分析仪)拥有电子和机械衰减器,可以结合使用两者来优化性能。
在这些情况下,机械衰减器只需进行细微的调整变得获得更出色的的结果,步进大约为1或2dB。
* 更改分辨带宽滤波器――按下分析仪的带宽滤波器键,可降低分辨率带宽。
注:由于分辨率带宽降低,所以扫描时间会增加。
扫描速度的降低,可以减少测量结果和测量速度的变化。
* 启动噪声校正――一旦启动噪声校正功能,分析仪将会进行一次扫描,以测量当前中心频率的内部本底噪声,并将在以后进行的扫描中从测量结果中减去该内部本底噪声。
这种方法能够显著改善ACLR,有时改善幅度高达5dB。
* 采用另一种测量方法。
除了使用默认的测量方法(集成带宽或IBW)之外,也可以采用滤波IBW方法。
该方法使用了更加陡降的截止滤波器。
虽然这种方法会降低功率测量结果的绝对精度,但是对ACLR结果没有不利影响。
通过结合使用这些方法,信号分析仪可以利用其嵌入式LTE应用程序自动优化ACLR测量,实现性能与速度的最佳搭配。
对于典型的ACLR测量,测量结果可能改善高达10dB或更多(图3)。
如果测量需要最高的性能,那么可以进一步调整分析仪设置。
本文小结
符合标准的频谱测量(例如ACLR)对于射频工程师开发下一代无线系统具有极其重要的作用。
然而使用LTE应用软件进行测量时,受多种因素的影响,这些测量非常复杂:邻近信道带宽的变化,发射滤波器的选择,不同带宽和不同干扰灵敏度的信道之间的射频变量的交互。
应对这一挑战的实用解决方案是使用安装有特定标准测量应用软件的频谱分析仪或信号分析仪。
此组合能够减少复杂测量中的错误,自动配置限制表和指定的测试装置,确保测量具有出色的可重复性。
使用分析仪优化技术可以进一步改善测量结果。
图3:此处显示的是使用优化设置后的安捷伦X系列信号分析仪获得的ACLR测量结果。
与图2使用嵌入式N9080A LTE测量应用软件获得的结果相比,图3中的ACLR实现了11dB的改善。
表1:LTE发射机设计的复杂问题。