dependence on photocatalytic activity of TiO2-x NX powders.
等离子体效应增强TiO2-SiO2-Ag复合薄膜的光吸收(英文)
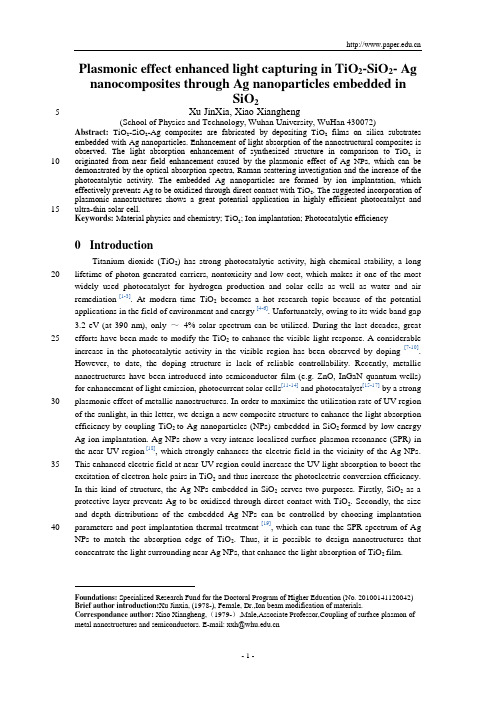
Plasmonic effect enhanced light capturing in TiO 2-SiO 2- Ag nanocomposites through Ag nanoparticles embedded inSiO 2Xu JinXia, Xiao Xiangheng5 (School of Physics and Technology, Wuhan University, WuHan 430072)Foundations: Specialized Research Fund for the Doctoral Program of Higher Education (No. 20100141120042) Brief author introduction:Xu Jinxia, (1978-), Female, Dr.,Ion beam modification of materials.Correspondance author: Xiao Xiangheng,(1979-),Male,Associate Professor,Coupling of surface plasmon of metal nanostructures and semiconductors. E-mail: xxh@Abstract: TiO 2-SiO 2-Ag composites are fabricated by depositing TiO 2 films on silica substrates embedded with Ag nanoparticles. Enhancement of light absorption of the nanostructural composites is observed. The light absorption enhancement of synthesized structure in comparison to TiO 2 is originated from near field enhancement caused by the plasmonic effect of Ag NPs, which can be 10demonstrated by the optical absorption spectra, Raman scattering investigation and the increase of the photocatalytic activity. The embedded Ag nanoparticles are formed by ion implantation, which effectively prevents Ag to be oxidized through direct contact with TiO 2. The suggested incorporation of plasmonic nanostructures shows a great potential application in highly efficient photocatalyst andultra-thin solar cell.15 Keywords: Material physics and chemistry; TiO 2; Ion implantation; Photocatalytic efficiency0 IntroductionTitanium dioxide (TiO 2) has strong photocatalytic activity, high chemical stability, a long lifetime of photon-generated carriers, nontoxicity and low cost, which makes it one of the most 20 widely used photocatalyst for hydrogen production and solar cells as well as water and air remediation [1-3]. At modern time TiO 2 becomes a hot research topic because of the potential applications in the field of environment and energy [4-6]. Unfortunately, owing to its wide band gap3.2 eV (at 390 nm), only ~ 4% solar spectrum can be utilized. During the last decades, great efforts have been made to modify the TiO 2 to enhance the visible light response. A considerable 25 increase in the photocatalytic activity in the visible region has been observed by doping [7-10]. However, to date, the doping structure is lack of reliable controllability. Recently, metallic nanostructures have been introduced into semiconductor film (e.g. ZnO, InGaN quantum wells) for enhancement of light emission, photocurrent solar cells [11-14] and photocatalyst [15-17] by a strong plasmonic effect of metallic nanostructures. In order to maximize the utilization rate of UV region 30 of the sunlight, in this letter, we design a new composite structure to enhance the light absorption efficiency by coupling TiO 2 to Ag nanoparticles (NPs) embedded in SiO 2 formed by low energy Ag ion implantation. Ag NPs show a very intense localized surface plasmon resonance (SPR) in the near-UV region [18], which strongly enhances the electric field in the vicinity of the Ag NPs. This enhanced electric field at near-UV region could increase the UV light absorption to boost the 35 excitation of electron-hole pairs in TiO 2 and thus increase the photoelectric conversion efficiency. In this kind of structure, the Ag NPs embedded in SiO 2 serves two purposes. Firstly, SiO 2 as a protective layer prevents Ag to be oxidized through direct contact with TiO 2. Secondly, the size and depth distributions of the embedded Ag NPs can be controlled by choosing implantation parameters and post-implantation thermal treatment [19], which can tune the SPR spectrum of Ag 40NPs to match the absorption edge of TiO 2. Thus, it is possible to design nanostructures that concentrate the light surrounding near Ag NPs, that enhance the light absorption of TiO 2 film.1 Experimental SectionHigh purity silica slides were implanted by Ag ions at 20, 40 and 60 kV to fluence of 5×1016 ions/cm 2 and at 40 kV to 1×1017 ions/cm 2 using a metal vapor vacuum arc ion source implanter, 45 respectively. The TiO 2-SiO 2-Ag nanostructural composites were obtained by depositing TiO 2 films (100 nm thick) on the surface of the as-implanted silica substrates using direct-current (DC) reactive magnetron sputtering system. For comparison, an un-implanted silica substrate was deposited with TiO 2 film under the same growth condition. Subsequently, all deposited samples were annealed at 500 °C in oxygen gas for 2 hours to obtain anatase phase TiO 2 film. The TiO 2 50covered silica substrates with embedded Ag NPs are named S1-S4 as shown in Table 1. The optical absorption spectra of all the samples were measured using a UV-vis-NIR dual-beam spectrometer (Shimadzu UV 2550) with wavelengths varied from 200 to 800 nm. Raman scattering spectra of all the samples were collected using a micro-Raman system (LabRAM HR800). An Ar laser (488.0 nm) was used as the excitation source, and the laser power was kept55 at 10 mW. The microstructure of the samples was investigated by using a JEOL JEM 2010 (HT) transmission electron microscopes (TEM) operated at 200 kV.Tab. 1 Ag ion implantation parameters for all samplessample Fluence of ion implantation(ions/cm2)Energy of ion implantation(kV)S1 5×1016 20S2 5×1016 40S3 1×1017 40 S4 5×1016 6060 2 Results and DiscussionThe photocatalytic efficiencies of TiO 2 and TiO 2-SiO 2-Ag nanostructural composites with an area of 4 cm 2 were evaluated by measuring the degradation rates of 5 mg/L methylene blue (MB) solution under UV-vis irradiation. A mercury lamp (OSRAM, 250 W with characteristic wavelength at 365 nm) was used as a light source. The TiO 2 and the TiO 2-SiO 2-Ag composites 65 films were placed into 40 mL MB solution with a concentration of 5 mg/L. Before irradiation, the samples were put into 40 mL MB solution for 30 minutes in the darkness to reach absorption equilibrium. The decolorization of MB solution was measured by an UV-vis spectrometer (Shimadzu UV 2550) at the wavelength of 664.0 nm. The absorption spectrum of the MB solution was measured at a time interval of 30 minutes and the total irradiation time was 4 hours. 70 Fig. 1 shows the optical absorption spectra of S1-S4 and the TiO 2 films. The absorption edge around 390 nm belongs to the intrinsic exciton absorption of TiO 2 [20]. The obvious absorption peaks at about 419-433 nm can be attributed to the SPR of Ag NPs formed by Ag ion implantation[21]. As seen the SPR of Ag NPs is close to the exciton edge (around 390 nm) of anatase TiO 2. Therefore, it is expected that an efficient energy transfer from the Ag NPs to TiO 2 can be occured. 75The position of Ag SPR absorption peak of the S2 is around 419 nm, which is blue shift comparing to that of the other three samples. The SPR peak of the S2 is the most close to the anatase TiO 2 exciton energy, therefore the strongest resonant coupling effect between Ag SPR and the excitons of the TiO 2 films maybe produce more effectively.80Fig. 1 The optical absorption spectra of S1-S4 and the pure TiO2 filmTo illustrate the strong near field induced by the SPR of Ag NPs, the Raman scattering spectra of S1-S4 and TiO2 are measured as presented in Fig. 2. The observed Raman bands at 144, 85199, 399, 516 and 640 cm-1 can be assigned to the Eg, Eg, B1g, A1g, or B1g and an Eg vibration modes of anatase phase, respectively, which are consistent with the characteristic patterns of pure anatase without any trace of rutile or brookite phase [22]. It is found that the Raman intensity for S1-S4 increase compared to that of TiO2, and the S2 shows the strongest Raman intensity. It is well known that Raman scattering intensity is proportional to the square of the electric field 90intensity [23], thus stronger Raman scattering attained from the TiO2-SiO2-Ag structure indicates that a stronger electric field is induced by Ag NPs embedded in SiO2 substrate. When the Ag NPs are irradiated by laser in spectral area of particle absorption band longer wavelength shoulder, a strong near field is produced due to the SPR, so Raman scattering is enhanced. As seen from Fig.2, the enhancement factors of Raman scattering of S1-S4 is different because of various coupling 95field efficiency. Thus, it is possible to conclude that the implantation energy and fluence have determined the Raman scattering enhancement factor.Fig. 2 The Raman scattering spectra of S1-S4 and the pure TiO2 filmTo understand the relationship between the size and depth distributions of Ag NPs in silica 100glass and the Raman scattering enhancement factor of the TiO2-SiO2-Ag nanocomposites, themicrostructural characterization of the S1-S4 were investigated by TEM as shown in Fig. 3. The TEM image of the S1 (Fig 3 (a)) shows that the size of Ag NPs appears to have a wide distribution.However, increasing the implantation energy to 40 kV as shown in Fig 3 (b), the Ag NPs in the S2 105are quite uniform in size (with an size of 20 nm) and distribute at nearly the same depth of 7 nm from the surface. Under high energy ion implantation, more heat will be induced in the sample in short time, which enhances the diffusion of Ag atoms. Therefore, the implanted Ag ions trend to aggregate to larger NPs around the projected range [24-26]. The near field induced by the SPR of Ag NPs is very strong due to the presence of the formed Ag NPs with bigger size and the near-field 110dipolar interactions between adjacent particles [27]. On the other hand, the dipolar interactions between adjacent particles with near the same size can result in a blue shift of SPR [28], thus the blue shift in the SPR peak of Ag NPs observed in the Fig. 1, which may produce a strongest resonant coupling effect between the SPR of Ag NPs and TiO2. It means the stronger near field can be induced. In this case, the S2 has the strongest Raman scattering enhancement factor. The 115size of Ag NPs in the S1 is smaller and the distribution is wider than that in the S2. It means that the near field induced by SPR of Ag NPs in the S1 is weaker than that in the S2. Further increasing the implantation energy to 60 kV as presented in Fig 3 (d), Ag NPs in the S4 reside deeper below the surface than that in the S2. Since the SP is an evanescent wave that exponentially decays with distance from the metal particles to the surface [29], the enhancement of 120Raman scattering decrease progressively with the increase of distance between Ag NPs with the TiO2 film, therefore Raman scattering intensity of the S4 has almost no enhancement. When the ion implantation fluence is increased to 1×1017 ions/cm2 with implantation energy of 40 kV (S3) as displayed in Figure 3 (c), large Ag NPs with size about 15 nm are formed near the surface and the small ones in deeper SiO2 matrix. The surface sputtering effect plays an important role for ion 125implantation at high fluence, the formed small Ag NPs near the surface are sputtered away by the subsequent implanted ions, as a result the large Ag NPs are populated near the surface of the S3[24].The Raman scattering enhancement factor is small with increasing the implantation fluence.Therefore, the Raman scattering enhancement demonstrates that the strong near field is actually induced by introducing Ag NPs, the increased field could locally concentrate the light surrounding the Ag NPs and thus enhance the absorption of light.130Fig. 3 Cross-sectional TEM images of the (a) S1, (b) S2, (c) S3, (d) S4.In order to study the enhancement of light absorption in TiO2-SiO2-Ag nanostructural 135composites, the photocatalysis activities of the S1-S4 are investigated by the UV degradation of MB solution at room temperature. For comparison, the TiO2 film is carried out under the same experimental conditions. As shown in Fig 4(a) inset one, the concentration of MB is decreased upon the irradiation time, and the TiO2 film can decompose 49% of MB after the UV irradiationfor 4 hours. However, the TiO 2-SiO 2-Ag nanostructural composite films obtained higherphotocatalytic efficiency than the pure TiO 2 film, and the S2 has the highest photocatalytic 140 efficiency than all other three samples, and degrade 72% MB. The enhancement ratio is as high as 47%. Meanwhile, the photodegradation of MB can be assumed to follow the classical Langmuir–Hinshelwood (L–H) kinetics [30], and its kinetics can be expressed as follows:0ln()A kt A = Where k is the apparent first-order reaction rate constant (min -1), 0A and A represent the145 absorbance before and after irradiation for time t , respectively. As displayed in Fig. 4(a), S2 shows the highest rate constant among all the samples, the k values of the S2 is about 2 times than that of the pure TiO 2. The kinetic rate constants follow the order S2>S3>S1>S4>TiO 2. This is consistent with the Raman scattering enhancement result.The near field enhancement in the TiO 2 layer due to the presence of the Ag NPs is also 150 simulated by Finite Difference Time Domain (FDTD) method as shown in Fig. 4(b). In our structure, we consider x as the light incident direction, the illuminating plane wave with a wavelength of 420 nm is y polarized, and an Ag NP with a diameter of 20 nm is embedded in SiO 2, and the distance to the surface of SiO 2 substrate is 7 nm. An amplitude enhancement to 3 can be observed. Theoretical and experimental results show that an enhancement of near field is induced 155by the SPR of Ag NPs. The SPR excitations cause a large increase in electromagnetic field in the vicinity of metal NPs, the localized amplification can increase the incident excitation field and boost the creation of hole-electron pairs, which results in the enhancement of the photocatalytic activity of TiO 2.160 Fig. 4 (a) The photodegradation of MB solution by S1-S4 and reference sample TiO 2 under UV light irradiation (inset one), and corresponding plots of 0ln()A Aversus the irradiation time, showing the linear fitting results; (b) amplitude enhancement of electric field inside a TiO 2 layer is simulated by FDTD method.3 Conclusion165 In conclusion, we have successfully demonstrated a plasmonic effect by simply incorporating Ag NPs with TiO 2 film. Optimum ion implantation conditions for Ag NPs synthesis in SiO 2 were experimentally estimated. The plasmonic effect occurring near interface of TiO 2 and silica glass has effectively enhanced the light trapping. Both the experimental data and the simulations show that the enhancement effect is attained from near field enhancement induced by the SPR of Ag 170 NPs. Our results have shown that the plasmonic effect has great potential in the application of increasing the UV light absorption in TiO 2 photocatalyst, and opening up opportunities for highly efficient ultra-thin film solar cells.References175[1] Wang D, Zou Y, Wen S and Fan D. A passivated codoping approach to tailor the band edges of TiO2 forefficient photocatalytic degradation of organic pollutants[J]. Appl. Phys. Lett. 2009, 95: 012106-1-3.[2] Han F, Kambala V S R, Srinivasan M, Rajarathnam D and Naidu R. Tailored titanium dioxide photocatalystsfor the degradation of organic dyes in wastewater treatment: A review[J]. Appl. Catal. A-Gen 2009, 359:25-40. 180[3] Yang J, You J, Chen C C, Hsu W C, Tan H R, Zhang X W, Hong Z and Yang Y. Plasmonic Polymer TandemSolar Cell[J]. ACS nano 2011, 5: 6210-6217.[4] Min B K, Heo J E, Youn N K, Joo O S, Lee H, Kim J H and Kim H S. Tuning of the photocatalytic1,4-dioxane degradation with surface Plasmon resonance of gold nanoparticles on titania[J]. Catal. Commun. 2009, 10:712 -715.185[5] Kumar M K, Krishnamoorthy S, Tan L K, Chiam S Y, Tripathy S and Gao H. Field Effects in PlasmonicPhotocatalyst by Precise SiO2 Thickness Control Using Atomic Layer Deposition[J]. ACS Catal. 2011, 1: 300-308.[6] Tong H, Quyang S, Bi Y, Umezawa N, Oshikiri M and Ye J. Nano- photocatalytic Materials: Possibilities andChallenges[J]. Adv. Mater. 2012, 24: 229-251.190[7] Anpo M. Preparation, Characterization, and Reactivities of Highly Functional Titanium Oxide-BasedPhotocatalysts Able to Operate under UV-Visible Light[J]. Bull. Chem. Soc. Jpn. 2004, 77:1427-1442.[8] Asahi R, Morikawa T, Ohwaki T, Aoki K and Taga Y. Visible-Light Photocatalysis in Nitrogen-DopedTitanium Oxides[J]. Science 2001, 293:269-271.[9] Ghicov A, Macak J M, Tsuchiya H, Kunze J, Haeublein V, Frey L and Schmuki P.Ion Implantation and 195Annealing for an Efficient N-Doping of TiO2 Nanotubes[J].Nano Lett.2006, 6 (5): 1080-1082.[10] Xu J H, Li J, Dai W L, Cao Y, Li H and Fan K. Simple fabrication of twist-like helix N,S-codoped titaniaphotocatalyst with visible-light response[J].Appl. Catal., B-Environ.2008, 79: 72-80.[11] Xiao X H, Ren F, Zhou X D, Peng T C, Wu W, Peng X N, Yu X F and Jiang C Z. Surface plasmon-enhancedlight emission using silver nanoparticles embedded in ZnO[J]. Appl. Phys. Lett.2010, 97:071909-1-3.200[12] Zhou X D, Xiao X H, Xu J X, Cai G X, Ren F, and Jiang C Z. Mechanism of the enhancement and quenchingof ZnO photoluminescence by ZnO-Ag coupling[J]. Europhys. Lett.2011, 93: 57009-p1-p6.[13] Zhang S G, Zhang X W, Yin Z G, Wang J X, Dong J J, Gao H L, Si F T, Sun S S and Tao Y. Localizedsurface plasmon-enhanced electroluminescence from ZnO-based heterojunction light-emitting diodes[J]. Appl.Phys. Lett.2011, 99: 181116-1-3.205[14] Okamoto K, Niki I, Shvartser A, Narukawa Y, Mukai T, and Scherer A. Surface-plasmon-enhanced lightemitters based on InGaN quantum wells[J]. Nature Mater.2004, 3: 601-605.[15] Awazu K, Fujimaki M, Rockstuhl C, Tominaga J, Murakami H, Ohki Y, Yoshida N, Watanabe T. APlasmonic Photocatalyst Consisting of Silver Nanoparticles Embedded in Titanium Dioxide[J]. J. Am. Chem.Soc.2008, 130: 1676 -1680.210[16] Oh J -H, Lee H, Kim D, Seong T Y. Effect of Ag nanoparticle size on the plasmonic photocatalytic propertiesof TiO2 thin films[J]. Surf. Coat. Technol. 2011, 206(1): 185-189.[17] Subrahmanyam A, Biju K P, Rajesh P, Jagadeesh Kumar K, Raveendra Kiran M. Surface modification ofsol gel TiO2 surface with sputtered metallic silver for Sun light photocatalytic activity: Initial studies[J]. Sol.Energy Mater. Sol. Cells 2012, 101: 241-248.215[18] Kerker M. The optics of colloidal silver: something old and something new[J]. J. Colloid Interface Sci.1985,105: 297-314.[19] Stepanov A L, Hole D E and Townsend P D. Modification of size distribution of ion implanted silvernanoparticles in sodium silicate glass using laser and thermal annealing[J]. Nucl. Instr. Meth. Phys. Res. B 1999, 149: 89-98.220[20] Linsebigler A L, Lu G Q and Yates Jr J T. Photocatalysis on TiO2 Surfaces: Principles, Mechanisms, andSelected Results[J]. Chem. Rev.1995, 95:735-758.[21] Ren F, Jiang C Z, Liu C, Fu D J and Shi Y. Interface influence on the surface plasmon resonance of Agnanocluster composite[J]. Solid State Commun. 2005, 135: 268-272.[22] Zhang W F, He Y L, Zhang M S, Yin Zand Chen Q. Raman scattering study on anatase TiO2 nanocrystals[J]. 225J. Phys. D: Appl. Phys. 2000, 33: 912-916.[23] Willets K A, Van Duyne R P. Localized Surface Plasmon Resonance Spectroscopy and Sensing[J]. Annu.Rev. Phys. Chem. 2007, 58: 267-297.[24] Ren F, Xiao X H, Cai G X, Wang J B, Jiang C Z. Engineering embedded metal nanoparticles with ion beamtechnology[J]. Appl. Phys. A.2009, 96: 317-325.230[25] Xiao X H, Ren F, Wang J B, Liu C, Jiang C Z. Formation of aligned silver nanoparticles by ionimplantation[J]. Mater. Lett.2007, 61: 4435-4437.[26] Ren F, Jiang C Z, Liu C, Wang J B and Oku T. Controlling the morphology of Ag nanoclusters by ionimplantation to different doses and subsequent annealing[J]. Phys. Rev. Lett. 2006, 97: 165501-1-4.[27] Biteen J S, Lewis N S, and Atwater H A. Spectral tuning of plasmon-enhanced silicon quantum dot 235luminescence[J]. Appl. Phys. Lett. 2006, 88:131109-1-3.[28] Maier S A and Atwater H A. Plasmonics Localization and guiding of electromagnetic energy inmetal/dielectric structures[J]. J. Appl. Phys. 2005, 98: 011101-1-10.[29] Chen C W, Wang C H, Wei C M, Chen Y F. Tunable emission based on the composite of Au nanoparticlesand CdSe quantum dots deposited on elastomeric film[J]. Appl. Phys. Lett. 2009, 94: 071906-1-3.240[30] Al-Ekabi H and Serpone N. kinetic studies in heterogeneous photocatalysis. 1. photocatalytic degradatlon ofchlorinated phenols in aerated aqueous solutions over TiO2 supported on a glass matrix[J]. J. Phys. Chem.1988, 92:5726-5731.等离子体效应增强TiO2-SiO2-Ag复合薄膜的光吸收徐进霞,肖湘衡245(武汉大学物理科学与技术学院,武汉 430072)摘要:利用离子注入方法将Ag离子注入到SiO2玻璃中,再利用反应磁控溅射沉积TiO2薄膜。
Photocatalysis A to Z — What we know and what we do not know in a scientific sense
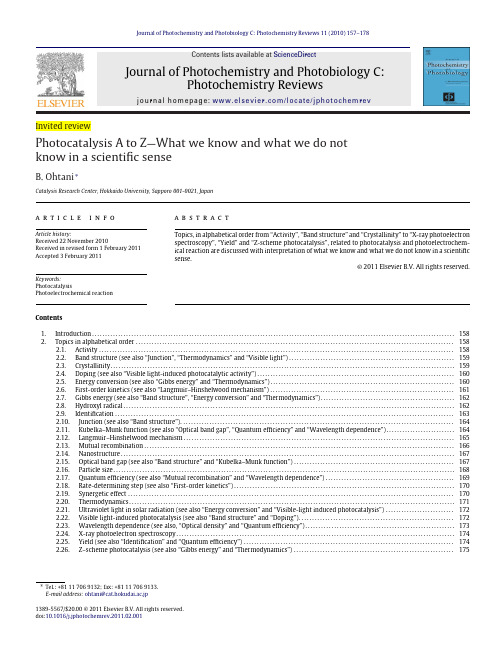
原位红外研究纳米管TiO2光催化降解乙醇
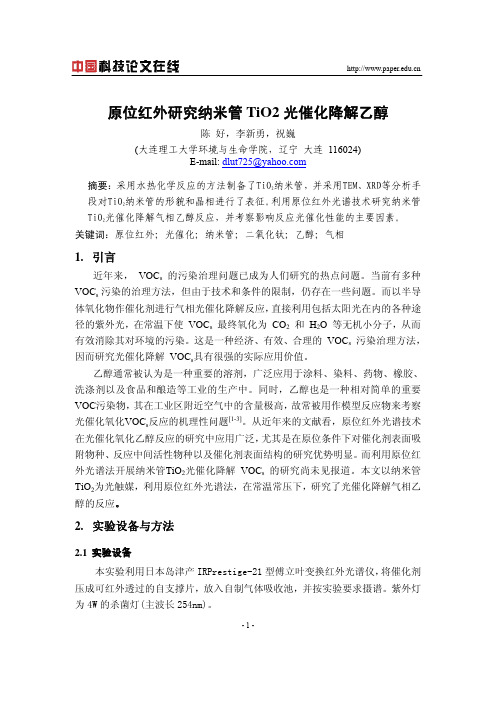
原位红外研究纳米管TiO2光催化降解乙醇陈 好,李新勇,祝巍(大连理工大学环境与生命学院,辽宁 大连 116024)E-mail: dlut725@纳米管,并采用TEM、XRD等分析手摘要:采用水热化学反应的方法制备了TiO2纳米管的形貌和晶相进行了表征。
利用原位红外光谱技术研究纳米管段对TiO2TiO光催化降解气相乙醇反应,并考察影响反应光催化性能的主要因素。
2关键词:原位红外;光催化;纳米管;二氧化钛;乙醇;气相1.引言近年来, VOC s的污染治理问题已成为人们研究的热点问题。
当前有多种VOC s 污染的治理方法,但由于技术和条件的限制,仍存在一些问题。
而以半导体氧化物作催化剂进行气相光催化降解反应,直接利用包括太阳光在内的各种途径的紫外光,在常温下使 VOC s最终氧化为 CO2和H2O 等无机小分子,从而有效消除其对环境的污染。
这是一种经济、有效、合理的 VOC s污染治理方法,因而研究光催化降解 VOC s具有很强的实际应用价值。
乙醇通常被认为是一种重要的溶剂,广泛应用于涂料、染料、药物、橡胶、洗涤剂以及食品和酿造等工业的生产中。
同时,乙醇也是一种相对简单的重要VOC污染物,其在工业区附近空气中的含量极高,故常被用作模型反应物来考察光催化氧化VOC s反应的机理性问题[1-3]。
从近年来的文献看,原位红外光谱技术在光催化氧化乙醇反应的研究中应用广泛,尤其是在原位条件下对催化剂表面吸附物种、反应中间活性物种以及催化剂表面结构的研究优势明显。
而利用原位红外光谱法开展纳米管TiO2光催化降解 VOC s的研究尚未见报道。
本文以纳米管TiO2为光触媒,利用原位红外光谱法,在常温常压下,研究了光催化降解气相乙醇的反应。
2.实验设备与方法2.1实验设备本实验利用日本岛津产IRPrestige-21型傅立叶变换红外光谱仪,将催化剂压成可红外透过的自支撑片,放入自制气体吸收池,并按实验要求摄谱。
紫外灯为4W的杀菌灯(主波长254nm)。
光电催化反应的英文

光电催化反应的英文## Photocatalytic reactions.Photocatalytic reactions are a type of chemical reaction that is initiated by the absorption of light. The absorbed light energy excites an electron in the photocatalyst, which then reacts with a substrate molecule to form a new product. Photocatalytic reactions are used in a variety of applications, including water purification,air pollution control, and solar energy conversion.The basic mechanism of a photocatalytic reaction is as follows:1. Light absorption: A photon of light is absorbed by the photocatalyst, exciting an electron from the valence band to the conduction band.2. Charge separation: The excited electron is separated from the hole that is created in the valence band.3. Redox reactions: The separated electron and holereact with substrate molecules to form new products.The efficiency of a photocatalytic reaction depends ona number of factors, including the following:The wavelength of light used.The intensity of light.The concentration of the photocatalyst.The concentration of the substrate.The temperature of the reaction.The pH of the reaction.Photocatalytic reactions can be used to accomplish a wide variety of chemical reactions, including the following:Water purification: Photocatalytic reactions can be used to remove organic contaminants from water.Air pollution control: Photocatalytic reactions can be used to remove nitrogen oxides and other pollutants fromthe air.Solar energy conversion: Photocatalytic reactions can be used to convert solar energy into electrical energy.Photocatalytic reactions are a promising technology for a variety of applications. They are efficient, environmentally friendly, and can be used to accomplish a wide variety of chemical reactions.### 中文回答:光电催化反应是一种由光吸收引发的化学反应。
Science-2001-Asahi-269-71
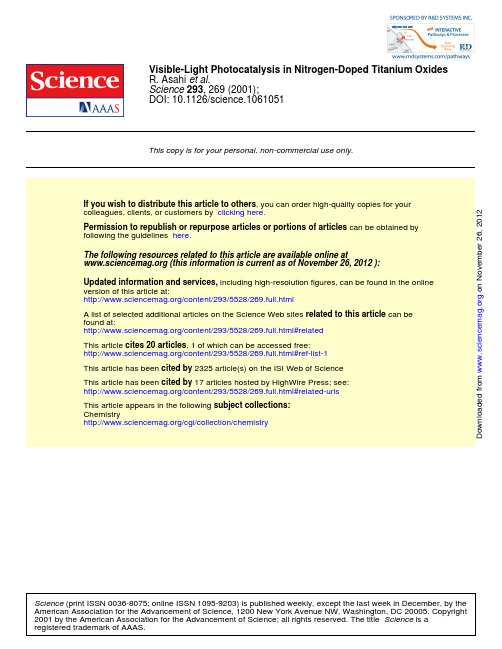
DOI: 10.1126/science.1061051, 269 (2001);293 Science et al.R. Asahi Visible-Light Photocatalysis in Nitrogen-Doped Titanium OxidesThis copy is for your personal, non-commercial use only.clicking here.colleagues, clients, or customers by , you can order high-quality copies for your If you wish to distribute this article to othershere.following the guidelines can be obtained by Permission to republish or repurpose articles or portions of articles): November 26, 2012 (this information is current as of The following resources related to this article are available online at/content/293/5528/269.full.html version of this article at:including high-resolution figures, can be found in the online Updated information and services, /content/293/5528/269.full.html#related found at:can be related to this article A list of selected additional articles on the Science Web sites /content/293/5528/269.full.html#ref-list-1, 1 of which can be accessed free:cites 20 articles This article 2325 article(s) on the ISI Web of Science cited by This article has been /content/293/5528/269.full.html#related-urls 17 articles hosted by HighWire Press; see:cited by This article has been/cgi/collection/chemistry Chemistrysubject collections:This article appears in the following registered trademark of AAAS.is a Science 2001 by the American Association for the Advancement of Science; all rights reserved. The title Copyright American Association for the Advancement of Science, 1200 New York Avenue NW, Washington, DC 20005. (print ISSN 0036-8075; online ISSN 1095-9203) is published weekly, except the last week in December, by the Science o n N o v e m b e r 26, 2012w w w .s c i e n c e m a g .o r g D o w n l o a d e d f r o m␣S,were obtained from nsbury [J.C.Rochet et al.,Biochemistry 39,10619(2000)].21.The silver-stained band at 22kD in an HP2A immu-noprecipitate of normal human brain and a co-mi-grating silver-negative slice from an adjacent lane of a parkin-preabsorbed HP2A precipitate were excised,trypsin digested and subjected to blind analysis by mass spectrometry at MDS Proteomics,Inc.(Toronto,ON)[A.Shevchenko et al.,Anal.Chem .68,850(1996)].The HP2A-specific 22-kD protein yielded tryptic peptides corresponding to aa 13–21,44–58,46–58,59–80,61–80,81–96,and 81–97of human ␣S (GenBank accession #L08850),each ending with a lysine,as expected.No ␣S sequence was detected in the co-migrating slice from the preabsorbed lane.22.Y.Mizuno,N.Hattori,H.Mori,Biomed.Pharmaco-ther.53,109(1999).23.H.Shimura et al.,Ann.Neurol.45,668(1999).24.N.Hattori et al.,Ann.Neurol.44,935(1998).25.A.L.Talis,J.M.Huibregtse,P.M.Howley,J.Biol.Chem.273,6439(1998).26.M.Scheffner,J.M.Huibregtse,R.D.Vierstra,P.M.Howley,Cell 75,495(1993).27.Y.Jiang,E.Lev-Lehman,J.Bressler,T.F.Tsai,A.L.Beaudet,Am.J.Hum.Genet.65,1(1999).28.Human UbcH7and UbcH8were expressed in M15E.coli harboring the Lac -repressor expressing plasmid pREP4,using the QIA express system (QIAGEN,Va-lencia,CA)with an NH 2-terminal 6xHis tag.Proteinswere purified on nickel NTA-agarose (QIAGEN).En-zymatic activity was tested in Ub shift assays.29.Parkin was immunoprecipitated (yielding IP-parkin)from frontal cortex homogenates or HEK293cells transiently transfected with myc-parkin cDNA (10g)(13).IP parkin was incubated at 37°C in 50l of reaction buffer containing ATP (4mM ATP in 50mM Tris-HCl,pH 7.5,2mM MgCl 2),100ng of recombi-nant human E1,2g of UbcH7(E2),and 2g His-Ub (all from Affinity Research Products,Exeter,UK).The reaction was terminated by adding 20l of 4X sample buffer,and 25l aliquots of the reaction mixtures were electrophoresed and immunoblotted.30.We prepared WT and ARPD-mutant parkin cDNAs [N.Hattori et al.,mun.249,754(1998);L.Terreni et al.,Neurology 56,463(2001)]in a pcDNA3.1(ϩ)vector using the QuikChange site-directed mutagenesis kit (Strat-agene,La Jolla,CA)and transiently transfected them into HEK293cells using Lipofectamine 2000(Gibco,Rockville,MD),as per manufacturers’instructions.31.For enzymatic digestion of HP2A precipitates,N -glycosidase,sialidase A,endo-O-glycosidase [with bovine fetuin as a control protein (ProZyme,San Leandro,CA)],and protein phosphatase-1(Sigma)were used as per manufacturers’instructions.32.C.A.Joazeiro,A.M.Weissman,Cell 102,549(2000).33.P.J.Kahle et al.,J.Neurosci.20,6365(2000).34.M.Ivan et al.,Science 292,464(2001).35.P.Jaakkola et al.,Science 292,468(2001).36.J.Q.Trojanowski,M.Goedert,T.Iwatsubo,V.M.Lee,Cell Death Differ.5,823(1999).37.M.S.Goldberg,nsbury,Nature Cell Biol.2,E115(2000).38.N.F.Bence,R.M.Sampat,R.R.Kopito,Science 292,1552(2001).39.C.J.Cummings et al.,Neuron 24,879(1999).40.L.Wells,K.Vosseller,G.W.Hart,Science 291,2376(2001).41.We thank H.Mori,Y.Mizutani,and K.Yamane for ARPDbrain specimens;J.Chan for providing normal human brain;and the patients’families for the donation of tissue.We also thank K.Wynn and P.Howley for anti-E6-AP (JH-16),T.Suzuki and K.Tanaka for a parkin cDNA-containing vector,nsbury for reagents and critical review of our manuscript,and M.Medina for experimental advice.H.S.is supported by a Pergolide Fellowship (Eli Lilly,Japan K.K.).M.G.S.is supported by the Grass Foundation (Robert S.Morison Fellowship),the Lefler Foundation,and the NIH (NS 02127).M.P.F.is supported by a Beeson Scholar Award.R.S.received grants from the National Bank of Austria.Supported by the Morris R.Udall Center of Excellence in PD (NS 38375)at Brigham and Women’s Hospital (M.P.F.,K.S.K.,and D.J.S.).12March 2001;accepted 6June 2001Published online 28June 2001;10.1126/science.1060627Include this information when citing this paper.Visible-Light Photocatalysis in Nitrogen-Doped TitaniumOxidesR.Asahi,*T.Morikawa,T.Ohwaki,K.Aoki,Y.TagaTo use solar irradiation or interior lighting efficiently,we sought a photocatalyst with high reactivity under visible light.Films and powders of TiO 2-x N x have revealed an improvement over titanium dioxide (TiO 2)under visible light (wavelength Ͻ500nanometers)in optical absorption and photocatalytic ac-tivity such as photodegradations of methylene blue and gaseous acetaldehyde and hydrophilicity of the film surface.Nitrogen doped into substitutional sites of TiO 2has proven to be indispensable for band-gap narrowing and photocat-alytic activity,as assessed by first-principles calculations and x-ray photo-emission spectroscopy.Since photoinduced decomposition of water on TiO 2electrodes was discovered (1),semicon-ductor-based photocatalysis has attracted exten-sive interest.One particular focus has been on applications in which organic molecules are photodegraded,such as water and air purifica-tions (2–4).Most of the investigations have focused on TiO 2(5–7),which shows relatively high reactivity and chemical stability under ul-traviolet (UV)light [wavelength ()Ͻ387nm],whose energy exceeds the band gap of 3.2eV in the anatase crystalline phase.The development of photocatalysts that can yield high reactivity under visible light (Ͼ380nm)should allow the main part of the solar spectrum,and even poor illumination of interior lighting,to be used.One approach has been to dope transition metals into TiO 2(8–10),and another has been to form reduced TiO x photo-catalysts (11,12).However,doped materials suffer from a thermal instability (9),an increase of carrier-recombination centers,or the require-ment of an expensive ion-implantation facility (10).Reducing TiO 2introduces localized oxy-gen vacancy states located at 0.75to 1.18eV below the conduction band minimum (CBM)of TiO 2(12),so that the energy levels of the optically excited electrons will be lower than the redox potential of the hydrogen evolution (H 2/H 2O)located just below the CBM of TiO 2(13)and that the electron mobility in the bulk region will be small because of the localization.We have considered whether visible-lightactivity could be introduced in TiO 2by doping,and we set the following requirements:(i)dop-ing should produce states in the band gap of TiO 2that absorb visible light;(ii)the CBM,including subsequent impurity states,should be as high as that of TiO 2or higher than the H 2/H 2O level to ensure its photoreduction ac-tivity;and (iii)the states in the gap should overlap sufficiently with the band states of TiO 2to transfer photoexcited carriers to reactive sites at the catalyst surface within their lifetime.Conditions ii and iii require that we use anionic species for the doping rather than cationic met-als,which often give quite localized d states deep in the band gap of TiO 2and result in recombination centers of carriers.We have cal-culated densities of states (DOSs)of the substi-tutional doping of C,N,F,P,or S for O in the anatase TiO 2crystal,by the full-potential lin-earized augmented plane wave (FLAPW)for-malism (14,15)in the framework of the local density approximation (LDA)(16)(Fig.1).The substitutional doping of N was the most effective because its p states contribute to the band-gap narrowing by mixing with O 2p states.Although doping with S shows a similar band-gap narrowing,it would be difficult to incorporate it into the TiO 2crystal because of its large ionic radius,as evidenced by a much larger formation energy required for the substi-tution of S than that required for the substitution of N (17).The states introduced by C and P are too deep in the gap to satisfy condition iii.The calculated imaginary parts of the dielectric functions of TiO 2-x N x indeed show a shift of the absorption edge to a lower energy by the N doping (Fig.2A)(18).Dominant transitions at the absorption edge have been identified withToyota Central R&D Laboratories,Nagakute,Aichi 480-1192,Japan.*To whom correspondence should be addressed.E-mail:rasahi@mosk.tytlabs.co.jpR E S E A R C H A R T I C L E S SCIENCE VOL 29313JULY 2001269o n N o v e m b e r 26, 2012w w w .s c i e n c e m a g .o r g D o w n l o a d e d f r o mthose from N 2p to Ti d xy ,instead of from O 2p as in TiO 2(19).Jansen and Letschert dem-onstrated tunable colorings in modified perov-skite oxinitrides,which suggested a similar band-gap control by N,although they did notaddress the detailed electronic states in these pigments (20).Photocatalysts for the present purpose,however,require more elaborate controls for doping besides the optical absorption;i.e.,conditions ii and iii.To this end,we have made a theoretical comparison among three systems:substitutional N doping,interstitial N doping,and both types of doping in the anatase TiO 2.In optimizing the positions of N in the eight TiO 2units per cell,we ob-served molecularly bonding states—NO and N 2—for the last two cases;the obtained bond lengths of N–O and N–N were 1.20and 1.16Å,respectively,which are compared with those of the NO molecule (1.15Å)and the N 2molecule (1.10Å).Such molecularly existing dopants give rise to the bonding states below the O 2p valence bands and antibonding states deep in the band gap (Fig.1B,N i and N i ϩs ).However,these states are well screened and hardly interact with the band states of TiO 2,and thus are unlikely to be effective for photocatalysis because of con-dition iii.The importance of substitutional site N doping is emphasized in the experi-mental results discussed below.We prepared TiO 2-x N x films by sputtering the TiO 2target in an N 2(40%)/Ar gas mixture.After being annealed at 550°C in N 2gas for 4hours,the films were crystalline,with features assignable to a mixed structure of the anatase and rutile crystalline phases,as determined by x-ray diffraction (XRD).The TiO 2-x N x films were yellowish and transparent.TiO 2films were prepared in a similar fashion by sputtering the TiO 2target in an O 2(20%)/Ar gas mixture and subsequently annealing it at 550°C in O 2gas for 4hours.XRD showed that the homo-geneous anatase crystalline phase was formed.Optical absorption spectra (Fig.2B)show that the TiO 2-x N x films noticeably absorb the light at less than 500nm,whereas the TiO 2films do not,which is in good agreement with the theo-retical results.Photocatalytic activity was evaluated by measuring decomposition rates of methylene blue as a function of the cutoff wavelength of the optical high-path filters under fluorescent light (Fig.3A).Substantial photocatalyticac-Fig.1.(A )Total DOSs of doped TiO 2and (B )the projected DOSs into the doped anion sites,calculated by FLAPW.The dopants F,N,C,S,and P were located at a substitutional site for an O atom in the anatase TiO 2crystal (the eight TiO 2units per cell).The results for N doping at an interstitial site (N i -doped)and that at both substitutional and interstitial sites (N i ϩs -doped)are also shown.The energy is measured from the top of the valence bands of TiO 2,and the DOSs for doped TiO 2are shifted so that the peaks of the O 2s states (at the farthest site from the dopant)are aligned with each other.Arb.unit,arbitraryunits.Fig.2.Optical proper-ties of TiO 2-x N x (thick lines)compared with TiO 2(thin lines).(A )Calculated imaginary parts of the dielectric functions (2),which are averaged over three (x ,y ,and z )po-larization vectors.(B )Experimental optical absorption spectra of TiO 2-x N x and TiO 2films.Fig.3.Photocatalytic proper-ties of TiO 2-x N x samples (solid circles)compared with TiO 2samples (open squares).(A )Decomposition rates [measur-ing the change in absorption of the reference light (⌬abs)]of methylene blue as a func-tion of the cutoff wavelength of the optical high-path filters under fluorescent light with the integrated photon flux of 2.45ϫ10Ϫ9einstein (E)s Ϫ1cm Ϫ2between 350and 520nm,compared with the re-sults under BL illuminationwith the integrated photon flux of 3.51ϫ10Ϫ9E s -1cm -2in the UV range.(B )CO 2evolution as a function of irradiation time (light on at zero)during the photodegradation of acetaldehyde gas [with an initial concentration of 485parts per million (ppm)]under UV irradiation (BL with a peak at 351nm and the light power of 5.4mW cm Ϫ2)and visible irradiation [fluorescent light cut by the optical high-path filter (SC42,Fuji Photo Film),with a peakintensity at 436nm and a light power of 0.9mW cm Ϫ2].(C )Contact angles of water as a function of time under interior lighting (with light powers of 28.5and 159.4W cm Ϫ2in the UV and visible ranges,respectively).All these light powers were measured by the UV radiometer (UVR-2,TOPCON,Tokyo,Japan)with detectors (UD-36for the UV range and UD-40for the visible range).13JULY 2001VOL 293SCIENCE 270 o n N o v e m b e r 26, 2012w w w .s c i e n c e m a g .o r g D o w n l o a d e d f r o mtivity under 500nm has been observed in TiO 2-x N x films,and the cutoff wavelength for photocatalytic activity corresponds well with the optical absorption spectra (21,22).Both TiO 2-x N x and TiO 2films reveal similar activity under UV light [represented by the results un-der black light (BL)illumination].We also evaluated the photodecomposition of gaseous acetaldehyde of TiO 2-x N x powder samples that were prepared by treating anatase TiO 2powder (ST01,Ishihara Sangyo Kaisha,Osaka,Japan)in the NH 3(67%)/Ar atmosphere at 600°C for 3hours.The Brunaer-Emmett-Teller surface areas of the TiO 2-x N x and TiO 2powders were measured as 67and 270m 2/g,respectively.Figure 3B shows CO 2concentra-tions,evolved as a result of the photodecompo-sition of acetaldehyde,as a function of irradia-tion time.The photocatalytic activity of the TiO 2-x N x sample is superior to that of the TiO 2sample in the visible range of irradiation,whereas both samples yield similar UV activity.We used the photodegradation process of acet-aldehyde proposed in (23)to estimate quantum yields for the CO 2evolution,based on the number of incident photons being 0.42%(TiO 2-x N x )and 0.14%(TiO 2)at 436nm,and 3.0%(TiO 2-x N x )and 2.6%(TiO 2)at 351nm.Our final evaluation of the photocatalytic activity was to measure contact angles of water on the sample films under interior lighting.The so-called photoinduced hydrophilic surface is known as an important application of TiO 2(24,25).In this experiment,we used the TiO 2-x N x and TiO 2films,on which SiO 2with a nominal thickness of 5nm was deposited to hold ad-sorbed water (25).The results show an excel-lent hydrophilic surface of the SiO 2/TiO 2-x N x film,which maintained a contact angle of 6°even after 30days,in contrast to the SiO 2/TiO 2film,whose contact angle constantly increased with time (Fig.3C).As with usual TiO 2spec-imens,our TiO 2-x N x samples resisted the attack of acid and alkaline solvents such as H 2SO 4,HCl,H 2O 2,and NaOH at the ambient temper-ature,and their photocatalytic performance wasstable during successive use under 100-W mer-cury lamp irradiation for more than 3months.To investigate N states in TiO 2-x N x ,we measured N 1s core levels with x-ray photo-emission spectroscopy (XPS).Three peak structures at the binding energies of 402,400,and 396eV were observed for the TiO 2-x N x films (Fig.4A).We observed similar XPS spectra for the TiO 2-x N x powder samples.The TiO 2films also included a small amount of N;however,the peak at 396eV was not observed.Saha et al .(26)investigated the N 1s XPS spectra during the oxidation process of TiN and assigned the peaks as atomic -N (396eV)and molecularly chemisorbed ␥-N 2(400and 402eV).To elucidate relations to photocatalytic activity systematically,we prepared TiO 2-x N x samples by annealing the TiO 2powder in the NH 3(67%)/Ar atmo-sphere at 550°to 600°C.The powder samples were used here,because the reaction temper-ature and the N concentration in the samples can be easily controlled by changing temper-ature and time of the NH 3treatment rather than by changing the sputtering conditions.Figure 4B shows the decomposition rates of methylene blue under visible light (Ͼ400nm)as a function of the ratio of the decom-posed area in the XPS peak at 396eV to the total area of N 1s .Each powder sample includ-ed the total N concentration of about 1atomic %.An increase of photocatalytic activity with an increase of the component of N with the XPS peak at 396eV is clearly observed.The decrease in photocatalytic activity observed in the powder sample (e)may be attributed to a change in the crystal structure of the sample caused by the high doping of N in the crystal,although we did not find a noticeable change in XRD.An optimum concentration of N with the XPS peak at 396eV,which may depend on the preparation process,was found at ϳ0.25atomic %(read from Fig.4B and the total concentra-tions of N in the samples)or TiO 1.9925N 0.0075.All these results,combined with the theoretical analyses described before (along with Fig.1),consistently show that the active sites of N for photocatalysis under visible light are the substi-tutional ones that can be identified with the atomic -N states peaking at 396eV in the XPS spectra.The active wavelength of TiO 2-x N x ,of less than 500nm,promises a wide range of applications,as it covers the main peak of the solar irradiation energy beyond Earth’s atmo-sphere (around 460nm)and an excellent light source peaking at 390to 420nm,provided by recently developed light-emitting indium gal-lium nitride diodes (27).References and Notes1.A.Fujishima,K.Honda,Nature 238,37(1972).2.M.A.Fox,M.T.Dulay,Chem.Rev.93,341(1993).3.M.R.Hoffmann et al.,Chem.Rev.95,69(1995).4.D.F.Ollis,H.Al-Ekabi,Eds.,Photocatalytic Purifica-tion and Treatment of Water and Air (Elsevier,Am-sterdam,1993).5.K.I.Hadjiivanov,D.K.Klissurski,Chem.Soc.Rev.25,61(1996).6.A.Heller,Acc.Chem.Res.28,503(1995).7.A.Linsebigler,G.Lu,J.T.Yates,Chem.Rev.95,735(1995).8.A.K.Ghosh,H.P.Maruska,J.Electrochem.Soc.124,1516(1977).9.W.Choi et al.,J.Phys.Chem.98,13669(1994),and references therein.10.M.Anpo,Catal.Surv.Jpn.1,169(1997).11.R.G.Breckenridge,W.R.Hosler,Phys.Rev.91,793(1953).12.D.C.Cronemeyer ,Phys.Rev.113,1222(1959).13.T.Sakata,T.Kawai,in Energy Resources Through Photochemistry and Catalysis ,M.Gra ¨tzel,Ed.(Aca-demic Press,New York,1983)pp.332–358.14.E.Wimmer et al .,Phys.Rev.B 24,864(1981).15.H.J.F.Jansen,A.J.Freeman,Phys.Rev.B 30,561(1984).16.L.Hedin,B.I.Lundqvist,J.Phys.C 4,2064(1971).17.The calculations have been done without any geom-etry optimization for the S doping,because the re-sulting atomic forces are too large to obtain reason-able positions in the present unit cell (the eight TiO 2units per cell),giving the positive formation energy of 4.1eV required for the substitution of S for O,compared with that of 1.6eV for the substitution of N.18.We used a rigid shift of the band gaps,1.14eV,for both TiO 2-x N x and TiO 2as in (19),assuming that the amount of the band-gap underestimation in LDA would not be affected by doping,because long-range screening properties in TiO 2-x N x are expected to be similar to those in TiO 2.19.R.Asahi et al.,Phys.Rev.B 61,7459(2000).20.M.Jansen,H.P.Letschert,Nature 404,980(2000).21.A possibility of the degradation of methylene blue through the so-called sensitization process in a visible range (Ͼ500nm)(22)can be negligible in the present measurement,because this influence,if any,would have appeared in the results equally at any cutoff wavelength of the high-path filter in the mea-surement range,which,however,we did not observe.22.K.Vinodgopal et al.,Environ.Sci.Technol.30,1660(1996).23.I.Sopyan et al.,J.Photochem.Photobiol.A Chem.98,79(1996).24.R.Wang et al .,Nature 388,431(1997).25.M.Machida et al.,J.Mater.Sci.34,2569(1999).26.N.C.Saha,H.G.Tompkins,J.Appl.Phys.72,3072(1992).27.N.M.Johnson et al.,Phys.Today 53,31(October 2000)and references therein.28.We thank A.J.Freeman and W.Mannstadt for their continuous support with the FLAPW code,and K.Tanaka and N.Isomura for their experimental sup-port.28December 2000;accepted 25May2001Fig.4.(A )N 1s XPS spectra of the (upper lines)TiO 2-x N x and (lower lines)TiO 2films.(B )Decomposi-tion rates (measuring the change in absorp-tion of the reference light after 10hours)of methylene blue in the aqueous solution un-der visible light (the same light source as the visible irradiation in Fig.3B)as a func-tion of the ratio of the decomposed area inthe XPS spectra with the peak at 396eV to the total area of N 1s .The total N concentrations for the powder samples were evaluated to be 1.0atomic %,a;1.1atomic %,b;1.4atomic %,c;1.1atomic %,d;and 1.0atomic %,e. SCIENCE VOL 29313JULY 2001271o n N o v e m b e r 26, 2012w w w .s c i e n c e m a g .o r g D o w n l o a d e d f r o m。
光电催化反应的英文
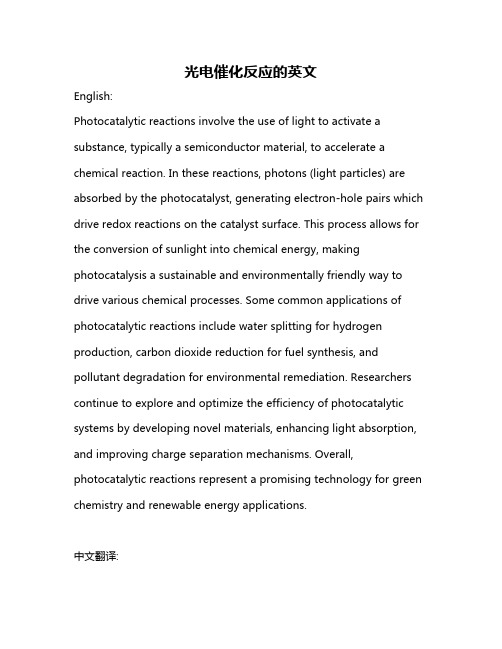
光电催化反应的英文English:Photocatalytic reactions involve the use of light to activate a substance, typically a semiconductor material, to accelerate a chemical reaction. In these reactions, photons (light particles) are absorbed by the photocatalyst, generating electron-hole pairs which drive redox reactions on the catalyst surface. This process allows for the conversion of sunlight into chemical energy, making photocatalysis a sustainable and environmentally friendly way to drive various chemical processes. Some common applications of photocatalytic reactions include water splitting for hydrogen production, carbon dioxide reduction for fuel synthesis, and pollutant degradation for environmental remediation. Researchers continue to explore and optimize the efficiency of photocatalytic systems by developing novel materials, enhancing light absorption, and improving charge separation mechanisms. Overall, photocatalytic reactions represent a promising technology for green chemistry and renewable energy applications.中文翻译:光催化反应涉及利用光激活物质,通常是半导体材料,加速化学反应。
Graphene-based semiconductor photocatalysts
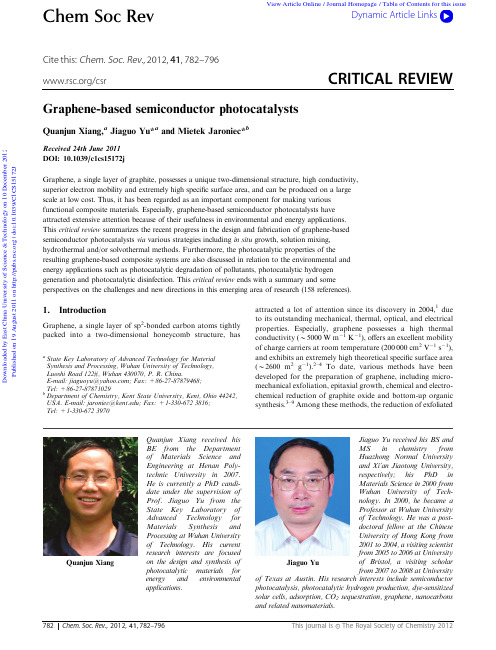
Cite this:Chem.Soc.Rev .,2012,41,782–796Graphene-based semiconductor photocatalystsQuanjun Xiang,a Jiaguo Yu*a and Mietek Jaroniec*bReceived 24th June 2011DOI:10.1039/c1cs15172jGraphene,a single layer of graphite,possesses a unique two-dimensional structure,high conductivity,superior electron mobility and extremely high specific surface area,and can be produced on a large scale at low cost.Thus,it has been regarded as an important component for making various functional composite materials.Especially,graphene-based semiconductor photocatalysts have attracted extensive attention because of their usefulness in environmental and energy applications.This critical review summarizes the recent progress in the design and fabrication of graphene-based semiconductor photocatalysts via various strategies including in situ growth,solution mixing,hydrothermal and/or solvothermal methods.Furthermore,the photocatalytic properties of theresulting graphene-based composite systems are also discussed in relation to the environmental and energy applications such as photocatalytic degradation of pollutants,photocatalytic hydrogen generation and photocatalytic disinfection.This critical review ends with a summary and someperspectives on the challenges and new directions in this emerging area of research (158references).1.IntroductionGraphene,a single layer of sp 2-bonded carbon atoms tightly packed into a two-dimensional honeycomb structure,hasattracted a lot of attention since its discovery in 2004,1due to its outstanding mechanical,thermal,optical,and electrical properties.Especially,graphene possesses a high thermal conductivity (B 5000W m À1K À1),offers an excellent mobility of charge carriers at room temperature (200000cm 2V À1s À1),and exhibits an extremely high theoretical specific surface area (B 2600m 2g À1).2–4To date,various methods have been developed for the preparation of graphene,including micro-mechanical exfoliation,epitaxial growth,chemical and electro-chemical reduction of graphite oxide and bottom-up organic synthesis.3–9Among these methods,the reduction of exfoliatedaState Key Laboratory of Advanced Technology for Material Synthesis and Processing,Wuhan University of Technology,Luoshi Road 122#,Wuhan 430070,P.R.China.E-mail:jiaguoyu@;Fax:+86-27-87879468;Tel:+86-27-87871029bDepartment of Chemistry,Kent State University,Kent,Ohio 44242,USA.E-mail:jaroniec@;Fax:+1-330-6723816;Tel:+1-330-6723970Quanjun XiangQuanjun Xiang received his BE from the Department of Materials Science and Engineering at Henan Poly-technic University in 2007.He is currently a PhD candi-date under the supervision of Prof.Jiaguo Yu from the State Key Laboratory of Advanced Technology for Materials Synthesis and Processing at Wuhan University of Technology.His current research interests are focused on the design and synthesis of photocatalytic materials for energy and environmental applications.Jiaguo YuJiaguo Yu received his BS and MS in chemistry from Huazhong Normal University and Xi’an Jiaotong University,respectively;his PhD in Materials Science in 2000from Wuhan University of Tech-nology.In 2000,he became a Professor at Wuhan University of Technology.He was a post-doctoral fellow at the Chinese University of Hong Kong from 2001to 2004,a visiting scientist from 2005to 2006at University of Bristol,a visiting scholar from 2007to 2008at Universityof Texas at Austin.His research interests include semiconductor photocatalysis,photocatalytic hydrogen production,dye-sensitized solar cells,adsorption,CO 2sequestration,graphene,nanocarbons and related nanomaterials.Chem Soc RevDynamic Article Links/csrCRITICAL REVIEWD o w n l o a d e d b yE a s t C h i n a U n i v e r s i t y o f S c i e n c e & T e c h n o l o g y o n 10 D e c e m b e r 2012P u b l i s h e d o n 19 A u g u s t 2011 o n h t t p ://p u b s .r s c .o r g | d o i :10.1039/C 1C S 15172JView Article Online / Journal Homepage / Table of Contents for this issuegraphene oxide (GO)was proven to be an effective and reliable method to produce graphene nanosheets owing to low cost and massive scalability.10Moreover,the surface properties of gra-phene can be adjusted via chemical modification,which offers tremendous opportunities for the development of functionalized graphene-based materials.8,11Such graphene-based materials show unique electronic and optical properties and good biocompatibility,which make these materials attractive for many potential applications including energy storage,12catalysis,13biosensors,14molecular imaging 15and drug delivery.16Recently,functionalized graphene-based semiconductor photocatalysts have attracted a lot of attention due to their good electron conductivity,large specific surface area and high adsorption.17–22Since the discovery of the photocatalytic splitting of water on TiO 2electrodes by Fujishima and Honda in 1972,23a significant progress has been made in the area of highly active oxide semiconductor photocatalysts because of their applic-ability in solar energy conversion and environmental protec-tion.Some semiconductors (e.g.,ZnO,WO 3,CdS,Bi 2WO 4and BiOCl,etc.)can act as photocatalysts for light-induced chemical transformations due to their unique electronic struc-ture composed of a filled valence band (VB)and an empty conduction band (CB).When a photon with energy of hv matches or exceeds the band gap energy (E g )of the semi-conductor,an electron in the VB is excited into the CB,leaving a positive hole in the VB.The photogenerated holes and electrons play a very important role in pollutant degradation and photocatalytic disinfection,and solar energy conversion including hydrogen production and solar photovoltaics,respectively.However,the photogenerated electrons and holes in the excited states are unstable and can easily recombine,dissipating the input energy as heat,which results in low efficiency of photocatalysis.24–27During the past decade,a variety of strategies have been employed to improve the photocatalytic performance ofsemiconductor photocatalysts,for example,via suitable textural design,28–33doping,34–37noble metal loading 38–40and forming semiconductor composites.41,42In particular,numerous attempts have been made to combine graphene with semiconductor photocatalysts to enhance their photo-catalytic performance.19–21,43–48For example,Kamat and co-workers 19,20,49,50produced GO–TiO 2nanocrystalline com-posites by sonicating dispersed TiO 2nanoparticles and GO in ethanol and demonstrated the feasibility of using graphene as an electron-transfer medium in the graphene/TiO 2composite photocatalysts.This pioneering work has stimulated an extensive research on the preparation,modification,and application of graphene-based semiconductor photocatalysts.Zhang et al.21reported graphene–P25TiO 2composites synthesized under hydrothermal conditions that exhibited an enhanced photocatalytic activity for the degradation of methylene blue in aqueous solutions.Ng et al.43demonstrated a great performance of the BiVO 4–GO composite photo-catalyst in the visible light range for water splitting.Since many important findings have been reported on the graphene-based semiconductor photocatalysts during the past few years,we believe that a comprehensive review on this subject is timely to promote further developments in this exciting area of research.This review is focused on the recent progress in the design,fabrication,modification and applications of graphene-based semiconductor photocatalysts,and provides some invigorating perspectives on the future developments.2.Preparation2.1Synthesis of grapheneSince the first report on graphene obtained by manual mechanical cleavage of graphite with a Scotch tape,1a wide range of techniques for the synthesis of this material have been reported.3In general,these techniques can be divided into ‘‘bottom-up’’and ‘‘top-down’’methods.In the bottom-up methods,graphene is synthesized from atoms or molecules via chemical reactions.Some typical examples are epitaxial growth on single-crystal SiC and chemical vapor deposition (CVD)on metal foil surfaces.5,6,51–53These resulting graphene films can be easily transferred to polymer substrates by etching away the metal supports.However,these methods are not widely used because of their complexity,limited scaling-up and high cost of the precious metal substrates.To date,high-quality graphene with a well-defined molecular structure was usually prepared by top-down methods such as chemical exfoliation of graphite,54,55thermal exfoliation 56and electrostatic deposition.57Especially,the most widely used technique is chemical reduction of GO as shown in Fig.1,which is usually conducted by Hummers’method.58According to this method,the reduced GO is prepared by exfoliating graphite oxide,obtained by oxidation of natural graphite powder with strong chemical oxidants,such as HNO 3,KMnO 4and H 2SO 4.The resulting GO product is usually purified by centrifugation,washing,and dialysis to remove some aggregates and various inorganic impurities such as residual ions and acids.More importantly,the exfoliated GO sheets usually possess a rich assortment of oxygen-containing groups,such as carboxylic,Mietek JaroniecMietek Jaroniec received his MS and PhD from M.Curie-Sklodowska Univer-sity,Poland,in 1972and 1976,respectively.Since 1991has been a Professor of Chemistry at Kent State University,Kent,Ohio (USA).Before joining Kent State he was a Professor of Chemistry at M.Curie-Sklodowska Univer-sity,Poland.His research interests revolve primarily around interdisciplinary topics of interfacial chemistry,chemical separations,andchemistry of materials,including physical adsorption at the gas/solid and liquid/solid interfaces,gas and liquid chromatography,adsorbents and catalysts.At Kent State he has established a vigorous research program in the area of ordered nanoporous materials such as ordered mesoporous silicas,organosilicas,inorganic oxides and carbons,focusing on their synthesis and environmental and energy-related applications.D o w n l o a d e d b yE a s t C h i n a U n i v e r s i t y o f S c i e n c e & T e c h n o l o g y o n 10 D e c e m b e r 2012P u b l i s h e d o n 19 A u g u s t 2011 o n h t t p ://p u b s .r s c .o r g | d o i :10.1039/C 1C S 15172Jhydroxyl,and epoxide functional groups.The presence of oxygen functionalities in GO allows interactions with the cations and provides reactive sites for the nucleation and growth of nanoparticles,which results in the rapid growth of various graphene-based composites.Moreover,the functional GO can be reduced to graphene with partial restoration of the sp 2-hybridized network by thermal,59chemical,60electrochemical,61photothermal,62photocatalytic,63sonochemical,64and micro-wave reduction methods.652.2Preparation of graphene-based semiconductor photocatalystsA variety of semiconductor photocatalysts have been used for the synthesis of graphene-based composites and/or functional GO.They mainly include metal oxides (e.g.TiO 2,18,21,66–74ZnO,48,75–80SnO 2,81–83Cu 2O,84Fe 2O 3,85NiO,86MnO 287and ZrO 288),salts (e.g.ZnS,89CdS,89–93CdSe,94Bi 2WO 6,95BiVO 4,43Sr 2Ta 2O 7,96ZnFe 2O 4,97InNbO 498and g -Bi 2MoO 699),metal-free polymers (e.g.graphitic carbon nitride 100,101)and silver/silver halides (Ag/AgCl,102,103and Ag/AgBr 103).The widely used preparation methods are in situ growth,solution mixing,hydrothermal and/or solvothermal method.The following paragraphs will give a more detailed description of the available synthesis routes.2.2.1In situ growth strategy.The direct growth strategy is widely used to prepare graphene based-metal compound composites.The most common precursors of graphene and metal compound are functional GO and metal salts,ually,the salt is mixed with GO and then converted to the corresponding oxide,forming a GO/metal compound com-posite.After reduction of GO,graphene based-metal compound composites were produced.For example,Sn 2+or Ti 3+ions were added into a functional GO dispersion solution and converted to SnO 2or TiO 2nanoparticles at low temperatures.In this process GO was reduced to graphene by SnCl 2or TiCl3.81The growth of SnO 2and TiO 2nanocrystals of different morphologies on the reduced GO sheets was attributed to the different reduction abilities and hydrolysis rates of Sn 2+and Ti 3+,respectively.A graphene–ZnOcomposite was synthesized analogously.Zn 2+ions were adsorbed on graphene oxide sheets and converted into ZnO nanoparticles with the addition of NaOH and NaBH 4under drying in air at 1501C.48After reduction of graphene oxide,a graphene/ZnO composite photocatalyst was produced.In this composite,the size of ZnO nanoparticles formed on graphene sheets was in the range of 10–20nm with a narrow particle size mbert et al.104also reported the in situ synthesis of composites comprised of flower-like anatase TiO 2–graphene oxide by the hydrolysis of TiF 4in the presence of aqueous dispersions of GO.In this case,when the concentration of graphene oxide was sufficiently high and stirring was off,a long-range ordered assemblies of TiO 2-GO sheets were obtained.Li et al.105developed a direct growth of uniform mesoporous anatase TiO 2nanospheres on graphene sheets by a template-free self-assembly process (see Fig.2).They used titanium sulfate and functional graphene sheets as the starting materials.The epoxy and hydroxyl functional groups on graphene sheets acted as heterogeneous nucleation sites by anchoring anatase nanoparticles,which resulted in the formation of well-dispersed mesoporous anatase TiO 2nanospheres on the graphene sheets.Interestingly,the oriented attachment of some very small primary TiO 2nanoparticles facilitated the formation of a single-crystal-like microstructure within a single nanosphere during the assembly process of these nano-spheres on graphene sheets.Further extensions of the direct growth strategy were used to prepare a variety of graphene based-composites.For example,Wang et al.106used anionic sulfate surfactants to assist the stabilization of graphene in aqueous solutions and to facilitate the self-assembly of in situ grown nanocrystalline TiO 2,rutile and anatase,with graphene.Park and co-authors 107prepared TiO 2nanoparticles coated with graphite oxide layers by the spontaneous exfoliation and reorganization of graphite oxides in aqueous slurry without using any chemical reagents under ambient conditions.Du et al.108also prepared the hierarchically ordered macro-mesoporous TiO 2–graphene composite film.They used a confinement self-assembly method to produce the hierarchicallyFig.1Preparation of graphene by chemical reduction of graphene oxide synthesized by Hummers’method.Reprinted with permission from ref.9.Copyright 2011,Wiley-VCH.Fig.2(a)Schematic illustration of the template-free self-assembly strategy for the preparation of mesoporous TiO 2nanospheres/graphene composites.(b)Side-view and (c)top-view SEM images of as-prepared mesoporous anatase TiO 2nanospheres/graphene compo-sites.Reprinted with permission from ref.105.Copyright 2011,Wiley-VCH.D o w n l o a d e d b yE a s t C h i n a U n i v e r s i t y o f S c i e n c e & T e c h n o l o g y o n 10 D e c e m b e r 2012P u b l i s h e d o n 19 A u g u s t 2011 o n h t t p ://p u b s .r s c .o r g | d o i :10.1039/C 1C S 15172Jordered porous titania films with two-dimensional hexagonal mesostructures and well-interconnected periodic macropores.Then,graphene was incorporated into the resulting titania framework by in situ reduction of graphene oxide added into the self-assembled system (see Fig.3).The in situ growth strategy can avoid the agglomeration of the semiconductor nanoparticles on the graphene sheets.Zhu et al.109reported a one-pot method for water-phase synthesis of high-quality graphene/TiO 2composite nanosheets using TiCl 3as a reducing agent and the titania precursor.Similarly,a graphene/CdS composite was also prepared by a one-step method using GO and Cd(CH 3COO)2Á2H 2O in DMSO.90During this one-step reaction,H 2S released from DMSO acted as both the reducing agent of GO and the sulfide source.Thus,the reduction of GO and the deposition of CdS on the graphene sheets occurred simultaneously,resulting in a uniform and tight distribution of CdS nanoparticles on graphene sheets.The size of the CdS nanoparticles in the composite was around 10nm (see Fig.4).Chen et al.110reported the fabrication of graphene sheets-wrapped anatase TiO 2hollow particles.The electroactive egg-like TiO 2hollow particles were first synthesized,and then these particles were functionalized with aminopropyltrieth-oxysilane to obtain a positively-charged surface.Next,the negatively charged GO sheets were linked to these functionalized TiO 2hollow particles via simple electrostatic interaction.Finally,the GO sheets were reduced to graphene sheets by thermal treatment under an inert atmosphere,leading to the formation of graphene–TiO 2composites.2.2.2Solution mixing method.Solution mixing has been widely used to fabricate graphene/semiconductor composite photocatalysts.For example,TiO 2particles and GO colloidshave been mixed ultrasonically,followed by ultraviolet (UV)-assisted photocatalytic reduction of GO to yield TiO 2/graphene composites.111Akhavan and Ghaderi 112used a similar strategy to prepare the TiO 2/graphene composite thin film.Paek et al.113synthesized the SnO 2sol by hydrolysis of SnCl 4with NaOH,and then the prepared graphene dispersion was mixed with the sol in ethylene glycol to form the SnO 2/graphene composite.Mukherji et al.96prepared nitrogen doped Sr 2Ta 2O 7coupled with graphene sheets by mixing the graphene oxide dispersion and Sr 2Ta 2O 7Àx N x ,followed by reduction of graphene oxide to graphene under xenon lamp irradiation.Zhu et al.103used the water/oil system to produce GO enwrapped Ag/AgX (X =Br,Cl)composites.They added aqueous solutions of GO and AgNO 3to chloroform solution of CTAB or CTAC under magnetic stirring at room temperature to produce Ag/AgX/GO hybrid composites (Fig.5).Considering graphene’s large network of sp 2hybridized carbon,this material can tend to form strong p –p bonds with other graphite-like materials.Sun et al.100synthesized a composite polymeric photocatalyst graphene/graphitic carbon nitride by a combined solution mixing–chemical reduction strategy.Graphitic carbon nitride was deposited on the surface of GO sheets to form a layered composite by polymerizing melamine molecules adsorbed on GO due to the p –p inter-action of aromatic structures between graphene oxide and graphitic carbon nitride.Similarly,Geng et al.94mixed graphene oxide sheets and the CdSe nanoparticles modified with pyridine to produce graphene–CdSe quantum dots composites.They thought that pyridine ligands could provide p –p interactions for the assembly of CdSe nanoparticles capped with pyridine on GO sheets.2.2.3Hydrothermal/solvothermal method.The hydro-thermal/solvothermal process,one of the traditional methods for crystal growth of semiconductors,is another effective method for the preparation of semiconductor composites with graphene.In this process,semiconductor nanoparticles or their precursors are loaded on the graphene oxide sheets,which are reduced to graphene.For example,Fu and Wang 97synthesized the ZnFe 2O 4–graphene composite photocatalyst with different graphene contents by a one-step hydrothermal method in ethanol-aqueous solution.They used Zn(NO 3)2Á6H 2O and Fe(NO 3)3Á9H 2O as precursors of ZnFe 2O 4,and GO as a source of graphene sheets.During the hydrothermalFig.3(a)Schematic illustration of the preparation of the ordered macro-mesoporous TiO 2–graphene composite film by in situ reduction of graphene oxide added into the self-assembly system.(b)SEM and (c)TEM images of the macro-mesoporous TiO 2–graphene composite film.Reprinted with permission from ref.108.Copyright 2011,American Chemical Society.Fig.4(a)TEM and (b)high-resolution TEM images of the graphene/CdS composite prepared by a solvothermal in situ growth method.Reprinted with permission from ref.90.Copyright 2010,Wiley-VCH.D o w n l o a d e d b yE a s t C h i n a U n i v e r s i t y o f S c i e n c e & T e c h n o l o g y o n 10 D e c e m b e r 2012P u b l i s h e d o n 19 A u g u s t 2011 o n h t t p ://p u b s .r s c .o r g | d o i :10.1039/C 1C S 15172Jreaction process,GO was reduced to graphene,and simulta-neously ZnFe 2O 4nanoparticles were formed on the graphene sheets (as shown in Fig.6).A graphene–Bi 2WO 6composite photocatalyst was also prepared by in situ hydrothermal reaction in the presence of GO.95Furthermore,some solvothermal experiments can result in the semiconductor nanoparticles of special morphology on graphene sheets.For example,Ding et al.114synthesized graphene-supportedultrathin anatase TiO 2nanosheets with exposed (001)high-energy facets by a simple solvothermal method.In this process,anatase TiO 2nanosheets directly grew onto the graphene oxide support during the solvothermal growth of TiO 2nanocrystals,and then GO was reduced to graphene via a thermal treatment under N 2/H 2,giving rise to the unique hybrid structure of the graphene–TiO 2composite.By using a different titanium source,Zhang et al.21synthesized the graphene–TiO 2nanocomposite photocatalyst by hydrothermal treatment of graphene oxide sheets and Degussa P25TiO 2powder in an ethanol–water solvent.In order to get a uniform mixture of graphene and the semiconductor photocatalyst,Wang et al.115used a one-step solvothermal method to produce graphene–TiO 2nanocomposites with well-dispersed particles by controlling the hydrolysis rate of titanium isopropoxide.Very recently,Shen et al.116found an environmentally friendly route for the preparation of graphene–TiO 2nanocomposites with a one-step hydrothermal method using glucose as the reducing agent.They claimed that this process is simple,scalable,and feasible because it utilizes only water and glucose.2.2.4Other methods.A nonaqueous atomic layer deposition (ALD)approach has been used to fabricate metal oxide–graphene hybrid nanocomposites.For example,Meng et al.83reported for the first time the preparation of the SnO 2–graphene nanocomposite using SnCl 4and H 2O as the ALD precursors.The SnO 2nano-particles/films as well as amorphous/crystalline phases were obtained by adjusting the growth temperature.Moreover,Du et al.88developed an electrochemical deposition method to prepare the graphene–ZrO 2composite on a glass carbon electrode.3.Photocatalytic applicationsIn recent years,semiconductor-mediated photocatalysis has attracted worldwide attention for its potential in environmental and energy-related applications.24,35,117–121However,the rapid recombination rate of photogenerated electron–hole pairs within photocatalytic materials results in its low efficiency,thus limiting its practical applications.Therefore,the suppression of recombination of charge carriers is the key for the enhancement of photocatalytic activity of semiconductor photocatalysts.Besides the conventional doping and adding co-sorbents,122carbon–semiconductor hybrid materials became a new class of photocatalysts,which recently has attracted a lot of attention.123–127Composites that combine carbon and semi-conductor photocatalysts could potentially offer desirable efficiency for separating electron–hole pairs.In this regard,graphene has been examined in combination with a semi-conductor photocatalyst,which resulted in improved photo-catalytic activity as shown in Table 1.As can be seen from this table the graphene-based semiconductor photocatalysts have been widely used for the degradation of pollutants,photo-catalytic hydrogen generation and photocatalytic disinfection,etc.In this section the main applications of graphene-based semiconductor photocatalysts are briefly summarized.3.1Photocatalytic degradation of pollutantsIn recent years,a great deal of effort has been devoted to solving the widespread pollution of effluents from urban andFig.5Typical SEM images of Ag/AgBr/GO (a)and Ag/AgCl/GO (b)composites prepared by a solution mixing method.Reprinted with permission from ref.103.Copyright 2011,American Chemical Society.Fig.6Typical TEM images of the ZnFe 2O 4/graphene composite photocatalyst prepared by an in situ hydrothermal method.The inset in (b)shows the high-resolution TEM images of the ZnFe 2O 4/graphene composite.Reprinted with permission from ref.97.Copyright 2011,American Chemical Society.D o w n l o a d e d b yE a s t C h i n a U n i v e r s i t y o f S c i e n c e & T e c h n o l o g y o n 10 D e c e m b e r 2012P u b l i s h e d o n 19 A u g u s t 2011 o n h t t p ://p u b s .r s c .o r g | d o i :10.1039/C 1C S 15172JTable 1Photocatalytic properties of graphene-semiconductor composite photocatalysts in comparison to the corresponding photocatalystsComposite photocatalyst Mass fraction of graphene Typical parameters ofphotocatalytic experiments Photocatalytic activity Reference photocatalyst;photocatalytic activity Enhancement factor over the reference photocatalyst Reference P25–G1.0wt%Decomposing methylene blue (MB)under UV light Degradation percentage (DP)of 85%P25or P25–CNTs;DP of 25%or 70%,respectively3.4or 1.221Decomposing MB under visible lightDP of 65%P25or P25–CNTs;DP of 12%or 52%,respectively5.4or 1.25P25–G 0.5wt%Decomposing benzene (gas phase)under UV light Conversion of6.4%in 28h P25;conversion of 1.2%in 28h5.31280.2wt%Decomposing MB under UV lightDP of 70%P25;DP of 60% 1.175.0wt%DP of 90% 1.530wt%DP of 58%0.970.2wt%Decomposing MB under visible lightDP of 40%P25;DP of 28% 1.45.0wt%DP of 65% 2.330wt%DP of 21%0.75TiO 2–GO No data Decomposing MB under UV lightDP of 99%in 15minP25;DP of 70%in 15min1.4129TiO 2–G film No data Decomposing 2,4-di-chlorophenoxyacetic acid under UV lightReaction rate:0.008min À1TiO 2film;reaction rate:0.002min À14.063TiO 2–G 10mg Decomposing MB under sunlight lightDP of 58%Pure TiO 2or P25;DP of 25%or 39%,respectively 2.3or 1.513030mg DP of 75% 3.0or 1.950mg DP of 72% 2.9or 1.8TiO 2–GO0.13wt%Decomposing methylorange (MO)under visible lightDP of 26%P25;DP of 22%1.181310.14wt%DP of 35% 1.590.25wt%DP of 22% 1.00.51wt%DP of 18%0.82TiO 2–G 0.6wt%Decomposing MB under UV lightReaction rate:0.071min À1TiO 2;reaction rate:0.045min À11.6108TiO 2–G No data Decomposing MO under UV lightDP of 85%Mixture of P25and graphene;DP of 45%1.89132TiO 2–G/MCM-410.05wt%Decomposing 2-propanol (gas phase)under UV lightConversion of 37%TiO 2/MCM-41;conversion of 26% 1.41330.15wt%Conversion of 45% 1.70.4wt%Conversion of 33% 1.270.6wt%Conversion of 25%0.96TiO 2–G 10wt%Decomposing rhodamine B (RhB)under UV light Reaction rate:0.20min À1Pure TiO 2or P25;reaction rate:0.05min À1or 0.068min À1,respectively4.0or 2.9134TiO 2–G 15wt%Decomposing RhB under visible light Reaction rate:0.0057min À1P25;reaction rate:0.0049min À11.281SnO 2–G 15wt%Reaction rate:0.011min À12.2TiO 2–GONo dataDecomposing MO under UV light Reaction rate:0.317min À1P25;reaction rate:0.0426min À17.467Photo-reductive Cr(VI)under UV light Conversion rate:0.0691min À1P25;conversion rate:0.0127min À1 5.4Bi 2WO 6–G No data Decomposing RhB under visible light DP of 90%in 4min Bi 2WO 6;DP of 70%in 8min>3.095ZnO–G0.1wt%Decomposing MB under UV light Reaction rate:0.047minÀ1ZnO;reaction rate:0.022min À1 2.1750.5wt%0.056min À1 2.51.0wt%0.069min À1 3.12.0wt%0.098min À1 4.53.0wt%0.091min À1 4.15.0wt%0.072min À13.3Ag/AgCl/GO 0.6wt%Decomposing MO under visible light DP of 71%Ag/AgX (X =Br,Cl);DP of 25% 2.8103Ag/AgBr/GO DP of 85% 3.4InNbO 4–GNo dataDecomposing MB under visible light Reaction rate:0.0346min À1InNbO 4;reaction rate:0.0185min À11.8798Decomposing 2,4-di-chlorophenol under visible lightReaction rate:0.0538minÀ1InNbO 4;reaction rate:0.0256min À1 2.1ZnFe 2O 4–G 20wt%Decomposing MB under visible lightNo data ZnFe 2O 4Enhancement 97Sr 2Ta 2O 7Àx N x –G 2.5wt%Photocatalytic H 2evolution under solar light;co-catalyst:Pt;sacrificialreagent:methanolR H 2:250m mol h À1Sr 2Ta 2O 7Àx N x ;R H 2:190m mol h À11.3965.0wt%293m mol h À1 1.510wt%110m mol h À10.58D o w n l o a d e d b yE a s t C h i n a U n i v e r s i t y o f S c i e n c e & T e c h n o l o g y o n 10 D e c e m b e r 2012P u b l i s h e d o n 19 A u g u s t 2011 o n h t t p ://p u b s .r s c .o r g | d o i :10.1039/C 1C S 15172J。
卤氧化铋在光催化领域的研究进展
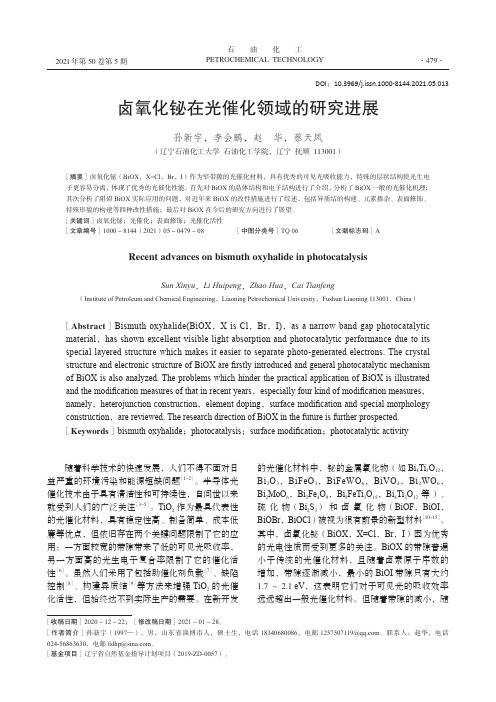
2021 年第50 卷第 5 期石油化工PETROCHEMICAL TECHNOLOGY·479·卤氧化铋在光催化领域的研究进展孙新宇,李会鹏,赵 华,蔡天凤(辽宁石油化工大学 石油化工学院,辽宁 抚顺 113001)[摘要]卤氧化铋(BiOX ,X=Cl ,Br ,I )作为窄带隙的光催化材料,具有优秀的可见光吸收能力,特殊的层状结构使光生电子更容易分离,体现了优秀的光催化性能。
首先对BiOX 的晶体结构和电子结构进行了介绍,分析了BiOX 一般的光催化机理;其次分析了阻碍BiOX 实际应用的问题,对近年来BiOX 的改性措施进行了综述,包括异质结的构建、元素掺杂、表面修饰、特殊形貌的构建等四种改性措施;最后对BiOX 在今后的研究方向进行了展望。
[关键词]卤氧化铋;光催化;表面修饰;光催化活性[文章编号]1000-8144(2021)05-0479-08 [中图分类号]TQ 06 [文献标志码]ARecent advances on bismuth oxyhalide in photocatalysisSun Xinyu ,Li Huipeng ,Zhao Hua ,Cai Tianfeng(Institute of Petroleum and Chemical Engineering ,Liaoning Petrochemical University ,Fushun Liaoning 113001,China )[Abstract ]Bismuth oxyhalide(BiOX ,X is Cl ,Br ,I),as a narrow band gap photocatalytic material ,has shown excellent visible light absorption and photocatalytic performance due to its special layered structure which makes it easier to separate photo-generated electrons. The crystal structure and electronic structure of BiOX are firstly introduced and general photocatalytic mechanism of BiOX is also analyzed. The problems which hinder the practical application of BiOX is illustrated and the modification measures of that in recent years ,especially four kind of modification measures ,namely ,heterojunction construction ,element doping ,surface modification and special morphology construction ,are reviewed. The research direction of BiOX in the future is further prospected.[Keywords ]bismuth oxyhalide ;photocatalysis ;surface modification ;photocatalytic activityDOI :10.3969/j.issn.1000-8144.2021.05.013[收稿日期]2020-12-22;[修改稿日期]2021-01-28。
溶胶_凝胶法制备TiO_2及其光催化性能研究
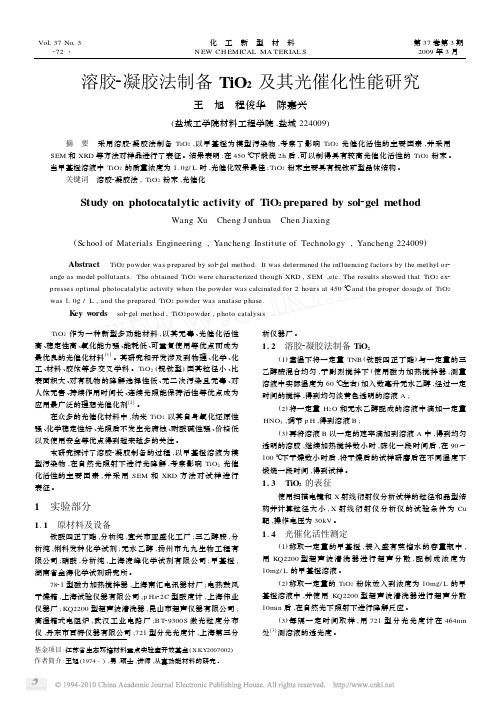
Vol 137No 13・72・化 工 新 型 材 料N EW CH EMICAL MA TERIAL S 第37卷第3期2009年3月基金项目:江苏省生态环境材料重点实验室开放基金(XKY2007002)作者简介:王旭(1974-),男,硕士,讲师,从事功能材料的研究。
溶胶2凝胶法制备TiO 2及其光催化性能研究王 旭 程俊华 陈嘉兴(盐城工学院材料工程学院,盐城224009)摘 要 采用溶胶2凝胶法制备TiO 2,以甲基橙为模型污染物,考察了影响TiO 2光催化活性的主要因素,并采用SEM 和XRD 等方法对样品进行了表征。
结果表明:在450℃下煅烧2h 后,可以制得具有较高光催化活性的TiO 2粉末。
当甲基橙溶液中TiO 2的质量浓度为1.0g/L 时,光催化效果最佳;TiO 2粉末主要具有锐钛矿型晶体结构。
关键词 溶胶2凝胶法,TiO 2粉末,光催化Study on photocatalytic activity of TiO 2prepared by sol 2gel methodWang Xu Cheng J unhua Chen Jiaxing(School of Materials Engineering ,Yancheng Instit ute of Technology ,Yancheng 224009)Abstract TiO 2powder was prepared by sol 2gel method.It was determined the influencing factors by the methyl or 2ange as model pollutants.The obtained TiO 2were characterized though XRD ,SEM ,etc.The results showed that TiO 2ex 2presses optimal photocatalytic activity when the powder was calcinated for 2hours at 450℃and the proper dosage of TiO 2was 110g /L ,and the prepared TiO 2powder was anatase phase.K ey w ords sol 2gel method ,TiO 2powder ,photo catalysis TiO 2作为一种新型多功能材料,以其无毒、光催化活性高、稳定性高、氧化能力强、能耗低、可重复使用等优点而成为最优良的光催化材料[1]。
2019年武汉理工大学材料学院博士研究生招生入围考试考生信息公示汇总表
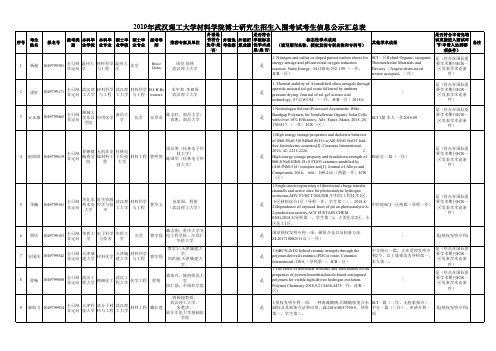
\
technology . 87:(1)83-94 (一作,JCR一区)2018.6
1.Nonhalogen Solvent-Processed Asymmetric Wide-
是
Bandgap Polymers for Nonfullerene Organic Solar Cells with Over 10% Efficiency, Adv. Funct. Mater, 2018, 28,
1049799924
全日制 非定向
天津科 技大学
高分子材 料与工程
武汉理 工大学
材料工程
戴红莲
武汉理工大学、 朱楚洪,
陆军军医大学基础医
学院
1.High energy storage properties and dielectric behavior
of (Bi0.5Na0.5)0.94Ba0.06Ti1-x(Al0.5Nb0.5)xO3 lead-
全日制 非定向
山东科 技大学
材料化学
山东科 技大学
材料加工 工程
韩颖超
王忠卫、曾荣昌 (山东科技大学)
nthanum magnesium hexaluminate thermal cycling lifetime
and CMAS corrosion behaviour,CERAMICS
INTERNATIONAL,2018年7月(一作,JCR一区)
2.Deposition and characterization of WC-Co hard-metal
coatings by high velocity oxy-fuel process combined with dry-
是(符合在国际重
photocatalysis英文解释
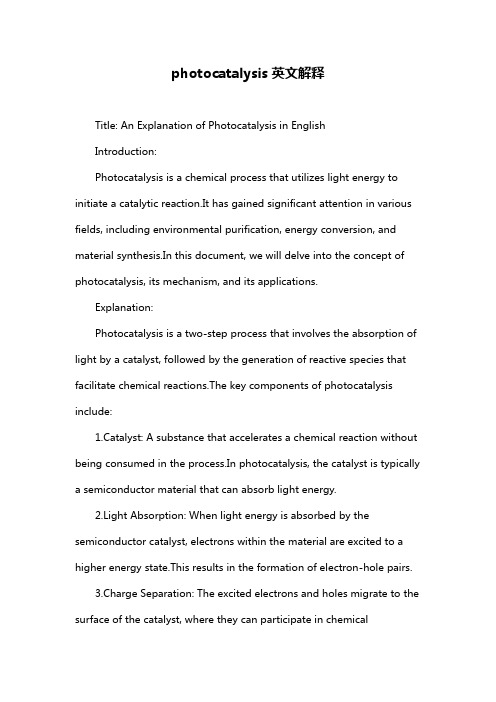
photocatalysis英文解释Title: An Explanation of Photocatalysis in EnglishIntroduction:Photocatalysis is a chemical process that utilizes light energy to initiate a catalytic reaction.It has gained significant attention in various fields, including environmental purification, energy conversion, and material synthesis.In this document, we will delve into the concept of photocatalysis, its mechanism, and its applications.Explanation:Photocatalysis is a two-step process that involves the absorption of light by a catalyst, followed by the generation of reactive species that facilitate chemical reactions.The key components of photocatalysis include:1.Catalyst: A substance that accelerates a chemical reaction without being consumed in the process.In photocatalysis, the catalyst is typicallya semiconductor material that can absorb light energy.2.Light Absorption: When light energy is absorbed by the semiconductor catalyst, electrons within the material are excited to a higher energy state.This results in the formation of electron-hole pairs.3.Charge Separation: The excited electrons and holes migrate to the surface of the catalyst, where they can participate in chemicalreactions.The separated charges are highly reactive and can interact with water, oxygen, or other molecules present in the system.4.Reactive Species Formation: The electrons and holes can react with water and oxygen to produce reactive species such as hydroxyl radicals (·OH) and superoxide anions (O2·-).These species have strong oxidative capabilities and can degrade organic pollutants, kill bacteria, or facilitate other chemical transformations.Applications of Photocatalysis:1.Environmental Purification: Photocatalysis is widely used for the degradation of harmful organic pollutants in water and air.It can break down persistent contaminants into harmless substances, thereby improving environmental quality.2.Self-Cleaning Surfaces: By applying a photocatalytic coating to surfaces, self-cleaning properties can be achieved.When exposed to light, the coating generates reactive species that decompose dirt, grime, and organic contaminants, keeping the surface clean.3.Antimicrobial Activity: Photocatalysis can be employed to kill or inhibit the growth of bacteria, viruses, and other microorganisms on surfaces.This is particularly useful in healthcare settings, where sterilization is crucial.4.Energy Conversion: Photocatalysis plays a vital role in splitting water into hydrogen and oxygen, a process known as watersplitting.This reaction is of great interest for renewable energy production, as hydrogen is a clean and efficient energy carrier.5.Material Synthesis: Photocatalysis is used in the synthesis of various materials, including nanoparticles, nanowires, and graphene.It offers precise control over the size, shape, and composition of the synthesized materials.In conclusion, photocatalysis is a powerful technique that harnesses light energy to drive chemical reactions.Its versatility and environmental friendliness make it an important area of research and application in numerous scientific and technological fields.。
光异构反应 释放氧离子

光异构反应释放氧离子英文回答:Heterogeneous photocatalysis is a process that involves the use of a photocatalyst to initiate a chemical reaction under light irradiation. This process is widely used in various applications, including environmental remediation, energy conversion, and organic synthesis. One of the key features of heterogeneous photocatalysis is the release of oxygen ions during the reaction.The release of oxygen ions in heterogeneous photocatalysis is primarily attributed to the oxidation of water molecules. In the presence of a suitable photocatalyst, such as titanium dioxide (TiO2), water molecules can be split into oxygen ions (O2-) and hydrogen ions (H+). This process is known as water oxidation and is a crucial step in the overall reaction.To illustrate this process, let's consider thephotocatalytic degradation of organic pollutants in water using TiO2 as the photocatalyst. When TiO2 is exposed to light, it absorbs photons and generates electron-hole pairs. The photogenerated holes (h+) on the surface of TiO2 can react with water molecules, leading to the formation of hydroxyl radicals (OH•) and oxygen ions (O2-):TiO2 + h+ + H2O → TiO2 + OH• + O2-。
纳米光催化材料的水热法合成
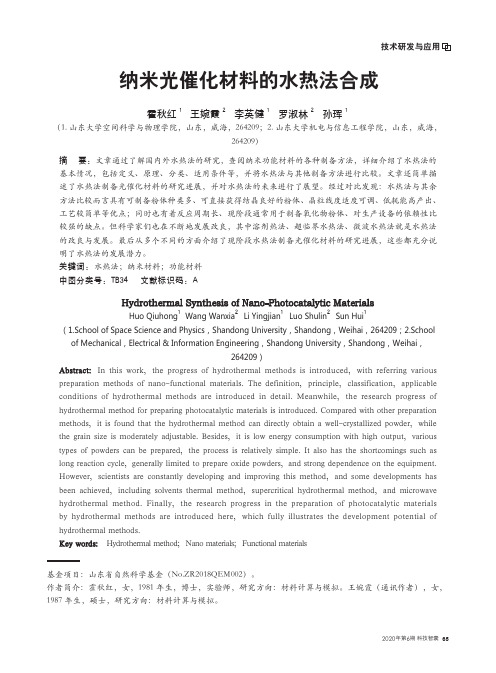
技术研发与应用摘 要:文章通过了解国内外水热法的研究,查阅纳米功能材料的各种制备方法,详细介绍了水热法的基本情况,包括定义、原理、分类、适用条件等,并将水热法与其他制备方法进行比较。
文章还简单描述了水热法制备光催化材料的研究进展,并对水热法的未来进行了展望。
经过对比发现:水热法与其余方法比较而言具有可制备粉体种类多、可直接获得结晶良好的粉体、晶粒线度适度可调、低耗能高产出、工艺较简单等优点;同时也有着反应周期长、现阶段通常用于制备氧化物粉体、对生产设备的依赖性比较强的缺点。
但科学家们也在不断地发展改良,其中溶剂热法、超临界水热法、微波水热法就是水热法的改良与发展。
最后从多个不同的方面介绍了现阶段水热法制备光催化材料的研究进展,这些都充分说明了水热法的发展潜力。
关键词:水热法;纳米材料;功能材料中图分类号:TB34 文献标识码:AHydrothermal Synthesis of Nano-Photocatalytic MaterialsHuo Qiuhong 1Wang Wanxia2Li Yingjian1Luo Shulin2Sun Hui1(1.School of Space Science and Physics,Shandong University,Shandong,Weihai,264209;2.School of Mechanical,Electrical & Information Engineering,Shandong University,Shandong,Weihai,264209)Abstract:In this work,the progress of hydrothermal methods is introduced,with referring various preparation methods of nano-functional materials. The definition,principle,classification,applicable conditions of hydrothermal methods are introduced in detail. Meanwhile,the research progress of hydrothermal method for preparing photocatalytic materials is introduced. Compared with other preparation methods,it is found that the hydrothermal method can directly obtain a well-crystallized powder,while the grain size is moderately adjustable. Besides,it is low energy consumption with high output,various types of powders can be prepared,the process is relatively simple. It also has the shortcomings such as long reaction cycle,generally limited to prepare oxide powders,and strong dependence on the equipment. However,scientists are constantly developing and improving this method,and some developments has been achieved,including solvents thermal method,supercritical hydrothermal method,and microwave hydrothermal method. Finally,the research progress in the preparation of photocatalytic materials by hydrothermal methods are introduced here,which fully illustrates the development potential of hydrothermal methods.Key words: Hydrothermal method;Nano materials;Functional materials纳米光催化材料的水热法合成霍秋红1 王婉霞2 李英健1 罗淑林2 孙珲1(1.山东大学空间科学与物理学院,山东,威海,264209;2.山东大学机电与信息工程学院,山东,威海,264209)基金项目:山东省自然科学基金(No.ZR2018QEM002)。
光催化co2反应器
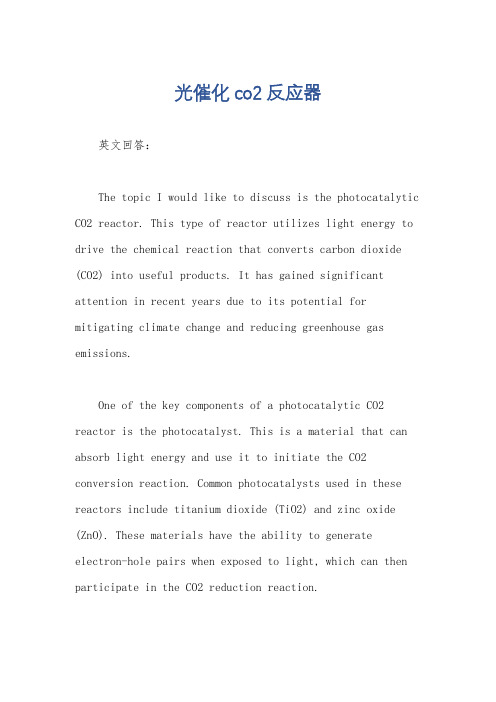
光催化co2反应器英文回答:The topic I would like to discuss is the photocatalytic CO2 reactor. This type of reactor utilizes light energy to drive the chemical reaction that converts carbon dioxide (CO2) into useful products. It has gained significant attention in recent years due to its potential for mitigating climate change and reducing greenhouse gas emissions.One of the key components of a photocatalytic CO2 reactor is the photocatalyst. This is a material that can absorb light energy and use it to initiate the CO2 conversion reaction. Common photocatalysts used in these reactors include titanium dioxide (TiO2) and zinc oxide (ZnO). These materials have the ability to generate electron-hole pairs when exposed to light, which can then participate in the CO2 reduction reaction.In addition to the photocatalyst, the reactor also requires a light source to provide the necessary energy for the photocatalytic reaction. This can be natural sunlightor artificial light sources such as LEDs. The choice oflight source depends on factors such as the desiredreaction rate and the availability of sunlight.The design of the reactor is another important aspectto consider. It should provide efficient light absorptionby the photocatalyst and ensure good contact between theCO2 and the photocatalyst surface. This can be achieved through various configurations such as slurry reactors,fixed-bed reactors, and fluidized-bed reactors.The photocatalytic CO2 reactor offers several advantages over traditional CO2 conversion methods. Firstly, it is a sustainable and environmentally friendly process as it utilizes renewable energy sources such as sunlight. Secondly, it can convert CO2 into valuable products such as fuels or chemicals, thereby reducing the dependence onfossil fuels. Finally, it has the potential to capture and utilize CO2 emissions from industrial processes, helping tomitigate climate change.To illustrate the concept further, let's consider an example. Imagine a large-scale photocatalytic CO2 reactor installed near a power plant. The reactor is equipped witha titanium dioxide photocatalyst and uses sunlight as the light source. As the sunlight hits the photocatalyst, it generates electron-hole pairs that react with CO2, converting it into a useful fuel such as methane. This fuel can then be used to generate electricity, creating aclosed-loop system that reduces CO2 emissions from thepower plant.中文回答:我想讨论的话题是光催化CO2反应器。
光催化全分解水
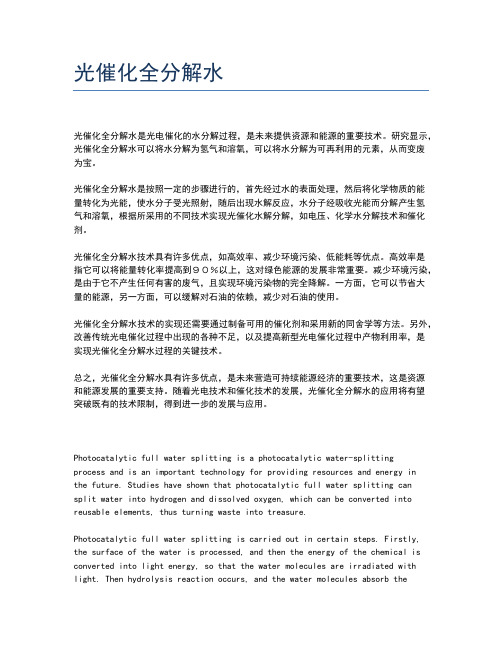
光催化全分解水光催化全分解水是光电催化的水分解过程,是未来提供资源和能源的重要技术。
研究显示,光催化全分解水可以将水分解为氢气和溶氧,可以将水分解为可再利用的元素,从而变废为宝。
光催化全分解水是按照一定的步骤进行的,首先经过水的表面处理,然后将化学物质的能量转化为光能,使水分子受光照射,随后出现水解反应,水分子经吸收光能而分解产生氢气和溶氧,根据所采用的不同技术实现光催化水解分解,如电压、化学水分解技术和催化剂。
光催化全分解水技术具有许多优点,如高效率、减少环境污染、低能耗等优点。
高效率是指它可以将能量转化率提高到90%以上,这对绿色能源的发展非常重要。
减少环境污染,是由于它不产生任何有害的废气,且实现环境污染物的完全降解。
一方面,它可以节省大量的能源,另一方面,可以缓解对石油的依赖,减少对石油的使用。
光催化全分解水技术的实现还需要通过制备可用的催化剂和采用新的同舍学等方法。
另外,改善传统光电催化过程中出现的各种不足,以及提高新型光电催化过程中产物利用率,是实现光催化全分解水过程的关键技术。
总之,光催化全分解水具有许多优点,是未来营造可持续能源经济的重要技术,这是资源和能源发展的重要支持。
随着光电技术和催化技术的发展,光催化全分解水的应用将有望突破既有的技术限制,得到进一步的发展与应用。
Photocatalytic full water splitting is a photocatalytic water-splittingprocess and is an important technology for providing resources and energy inthe future. Studies have shown that photocatalytic full water splitting cansplit water into hydrogen and dissolved oxygen, which can be converted into reusable elements, thus turning waste into treasure.Photocatalytic full water splitting is carried out in certain steps. Firstly, the surface of the water is processed, and then the energy of the chemical is converted into light energy, so that the water molecules are irradiated with light. Then hydrolysis reaction occurs, and the water molecules absorb thelight energy and decompose to produce hydrogen and dissolved oxygen. Depending on the different technologies used, photocatalytic hydrolysis can be achieved, such as voltage, chemical hydrolysis technology, and catalysts.Photocatalytic full water splitting technology has many advantages, such as high efficiency, reduced environmental pollution, low energy consumption, etc. High efficiency means that it can increase the energy conversion rate to more than 90%, which is very important for the development of green energy. Reducing environmental pollution is due to the fact that it does not generate any harmful exhaust gas, and complete degradation of pollutants can be achieved. On the one hand, it can save a lot of energy, on the other hand, it can reduce dependence on oil and reduce the use of oil.The realization of photocatalytic full water splitting also requires the preparation of available catalysts and the adoption of new reactions. In。
人们是否对科技产生依赖英语作文
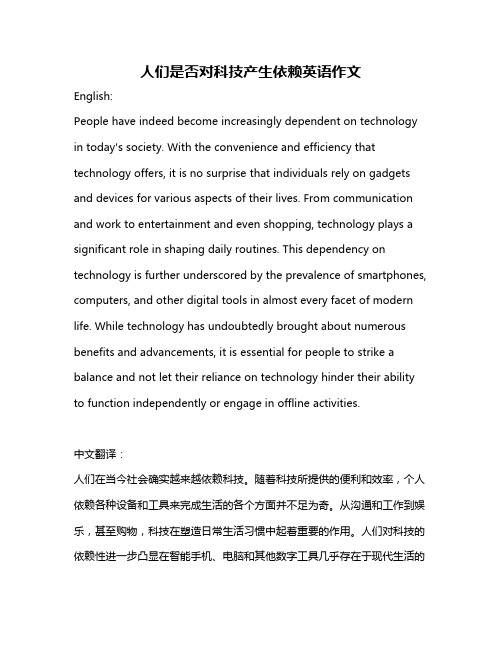
人们是否对科技产生依赖英语作文English:People have indeed become increasingly dependent on technology in today's society. With the convenience and efficiency that technology offers, it is no surprise that individuals rely on gadgets and devices for various aspects of their lives. From communication and work to entertainment and even shopping, technology plays a significant role in shaping daily routines. This dependency on technology is further underscored by the prevalence of smartphones, computers, and other digital tools in almost every facet of modern life. While technology has undoubtedly brought about numerous benefits and advancements, it is essential for people to strike a balance and not let their reliance on technology hinder their ability to function independently or engage in offline activities.中文翻译:人们在当今社会确实越来越依赖科技。
光合作用对于生态系统的意义英语作文

The Significance of Photosynthesis in Ecosystems Photosynthesis is a fundamental process that takes place in the cells of plants, algae, and some bacteria, enabling them to convert sunlight into energy-rich organic compounds. This process has significant implications for ecosystems and plays a crucial role in maintaining the balance of life on Earth.One of the primary significance of photosynthesis in ecosystems is the production of oxygen. During photosynthesis, plants utilize sunlight to convert carbon dioxide and water into glucose and oxygen. This oxygen is released into the atmosphere, supporting the respiration of many organisms, including humans. Without photosynthesis, the production of oxygen would significantly decrease, leading to a decline in the overall health of ecosystems.Furthermore, photosynthesis is the base of the food chain in most ecosystems. Autotrophic organisms, such as plants and algae, are capable of photosynthesis and serve as the primary producers in ecosystems. They form the foundation of the food chain by converting sunlight into energy-rich organic compounds that are essential for the growth and development of other organisms. Heterotrophs, including animals and some microorganisms, depend on these primary producers for their energy needs, highlighting the critical role of photosynthesis in sustaining the trophic structure of ecosystems.In addition to oxygen production and energy transfer, photosynthesis also plays a vital role in the global carbon cycle. By converting carbon dioxide into organic compounds, plants sequester carbon from the atmosphere, helping to mitigate the effects of climate change. This process not only regulates the amount of carbon dioxide in the atmosphere but also provides the necessary building blocks for the growth and development of living organisms.Moreover, photosynthesis contributes to the overall biodiversity of ecosystems. Plants that undergo photosynthesis provide habitats and food sources for a wide variety of organisms, contributing to the rich diversity of life forms on Earth. The ability of plants to harness sunlight and convert it into usable energy has allowed for the development of complex and diverse ecosystems that are essential for supporting life in all its forms.Given the significance of photosynthesis in ecosystems, it is essential to recognize the critical role that this process plays in maintaining the balance and sustainability of life on Earth. By understanding the importance of photosynthesis, we can appreciate the interconnectedness of all living organisms and work towards preserving and protecting the natural environments that support the intricate web of life on our planet.。
光敏化学作用 英文

光敏化学作用英文英文回答:Photochemical Action.Photochemical action refers to the chemical reactions that are initiated or accelerated by light. These reactions involve the absorption of light energy by a molecule, which then undergoes a chemical transformation. Photochemical reactions are important in a wide variety of natural and industrial processes, including photosynthesis, vision, and the production of vitamin D.The basic principles of photochemical action are relatively simple. When a molecule absorbs light, its electrons are excited to a higher energy level. This excited state is unstable, and the molecule will quickly return to its ground state by emitting a photon of light or by undergoing a chemical reaction. The type of chemical reaction that occurs will depend on the specific moleculeand the wavelength of light that is absorbed.Photochemical reactions can be classified into two main types: unimolecular and bimolecular. Unimolecular reactions involve the absorption of light by a single molecule, which then undergoes a chemical transformation. Bimolecular reactions involve the absorption of light by two molecules, which then react with each other.Photochemical reactions are used in a wide variety of applications. In photosynthesis, light energy is used to convert carbon dioxide and water into glucose and oxygen. In vision, light energy is used to activate rhodopsin, a protein that triggers the electrical signals that are sent to the brain. In the production of vitamin D, light energy is used to convert 7-dehydrocholesterol into vitamin D3.Photochemical reactions are also used in a variety of industrial processes. For example, photochemical reactions are used to produce plastics, dyes, and pharmaceuticals. Photochemical reactions are also used in the purification of water and air.中文回答:光敏化学作用。
Ag2CO3的制备、表征及其光催化性能

Ag2CO3的制备、表征及其光催化性能汪佳凤;桂志萍;司桂福;贾勇;周双生【摘要】以AgNO3和Na2CO3为原料通过沉淀法制备了Ag2CO3光催化剂,采用X-射线衍射(XRD)、扫描电镜(SEM)、傅里叶变换红外光谱(FTIR)和紫外可见光谱(UV-Vis)对所制备的样品进行了表征,并考察了该光催化剂在紫外光照射下对亚甲基蓝(MB)的催化降解效果.结果表明,Ag2CO3的禁带宽度约为2.53 eV,经60 min紫外光催化反应,0.5 g·L-1Ag2CO3对40 mg· L-1的MB溶液的降解率达到97.7%,比同等条件下TiO2的降解率高出31.1%.【期刊名称】《合肥师范学院学报》【年(卷),期】2016(034)003【总页数】4页(P45-48)【关键词】碳酸银;光催化剂;亚甲基蓝【作者】汪佳凤;桂志萍;司桂福;贾勇;周双生【作者单位】安徽中医药大学药学院,安徽合肥230012;绿色高分子材料安徽省重点实验室,安徽合肥230601;安徽中医药大学药学院,安徽合肥230012;安徽中医药大学药学院,安徽合肥230012;安徽中医药大学药学院,安徽合肥230012;安徽中医药大学药学院,安徽合肥230012;绿色高分子材料安徽省重点实验室,安徽合肥230601【正文语种】中文【中图分类】G61随着染料工业的迅速发展,染料废水的排放量日益增加,导致环境污染不断恶化,解决染料废水的降解问题越来越引起人们的广泛关注。
近年来,光催化降解技术因其绿色环保、价廉高效已成为有机污染物降解和净水处理方面的重要方法。
TiO2是目前应用最为广泛的金属氧化物半导体催化剂,具有化学稳定性好、耐光腐蚀、对有机污染物选择性小和矿化程度高等优点[1]。
然而,TiO2的光生电子和空穴易复合,导致量子产率低,制约着TiO2光催化效率的大幅提高[2]。
因此,探索和开发高效的新型光催化剂是当今光催化领域的重要发展方向。
- 1、下载文档前请自行甄别文档内容的完整性,平台不提供额外的编辑、内容补充、找答案等附加服务。
- 2、"仅部分预览"的文档,不可在线预览部分如存在完整性等问题,可反馈申请退款(可完整预览的文档不适用该条件!)。
- 3、如文档侵犯您的权益,请联系客服反馈,我们会尽快为您处理(人工客服工作时间:9:00-18:30)。
Nitrogen-Concentration Dependence on Photocatalytic Activity of TiO 2-x N x PowdersHiroshi Irie,Yuka Watanabe,and Kazuhito Hashimoto*Research Center for Ad V anced Science and Technology,The Uni V ersity of Tokyo,4-6-1Komaba,Meguro-ku,Tokyo 153-8904,Japan Recei V ed:January 31,2003Theof theTiO 2-with low nitrogen concentrations (<0.02)was evaluated by the of gaseous (IPA)under the same absorbed photon number,1.4×1014quanta ‚cm of visible (Vis)(UV)light.Regardless of the x value,the quantum yield values from irradiating with Vis light was lower than with UV light,which suggests that the isolated narrow band formed above the valence band is responsible for the Vis light response in the present nitrogen doped TiO 2.In addition,increasing the nitrogen concentration when irradiating with UV light lowered the quantum yields,indicating that the doping sites could also serve as sites.1.IntroductionTitanium dioxide (TiO 2)is an efficient photocatalyst 1-3but is activated only by irradiating with ultraviolet (UV)light.A current area of research in this field is to modify TiO 2so that it is sensitive to visible (Vis)light.One approach is to substitute Cr,Fe,or Ni,etc.,for a Ti site.4-6Anpo et al .5,6substituted Cr 3+or V 3+(V 4+)at lattice positions of Ti 4+in TiO 2by a metal ion-implantation method.They showed that the absorption band of the Cr 3+-doped TiO 2shifted to the visible light region and that irradiating with Vis light (wavelength >450nm)photo-catalytically decomposed NO into N 2,O 2,and N 2O.Another approach is to form Ti 3+sites by introducing an oxygen vacancy in TiO 2.7,8Ando et al .8reported the “oxygen vacancy-typed visible light sensitive TiO 2”anatase TiO 2was fabricated and treated under a plasma (H 2plasma).In 1986,Sato 9reported that the of NH 4Cl or NH 4-OH involving titanium hydroxide caused the photocatalytic sensitization of TiO 2into the Vis light region.He concluded that powders were NO x -doped TiO 2and that the of these TiO 2powders was due to the NO x Similarly,Noda et al .10prepared yellow-colored anatase TiO 2powders from a (NH 2)2‚H 2O and TiCl 4solution and concluded that the Vis light absorption was due to the oxygen vacancies.Recently,several groups reported Vis light sensitive TiO 2-based powders and thin films.11-14Sakatani et al.11prepared nitrogen-doped TiO 2powders by a hydrolytic process,using a TiCl 3solution and an ammonia solution,and reported that irradiating with Vis light in the presence of the powdered photocatalyst caused the photocatalytic decomposition of acetaldehyde to CO 2.They concluded that the nitrogen atoms (or ions)were doped at the interstitial sites and that these interstitial nitrogens were responsible for the Vis light response.Ihara et al .12also prepared a Vis light sensitive TiO 2photo-catalyst by a hydrolytic process,using a Ti(SO 4)2solution and an ammonia solution.They found that irradiating with Vis light in the presence of the prepared powder caused the photocatalytic decomposition of 2-propanol to CO 2and asserted that the oxygen vacancies were responsible for the Vis light response.Sakatani et al.and Ihara et al.insisted on a different conclusion for Vis light response,although they reported similar fabrication methods for Vis light sensitive TiO 2-based photocatalysts.Asahi et al.13,14theoretically calculated the band structure of the nitrogen-doped TiO 2and its Vis light induced photocatalysis for powders and thin films.Asahi insisted that the oxygen sites were substituted by nitrogen atoms and that these nitrogens were responsible for the Vis light sensitivity.In addition,Asahi concluded that the Vis light sensitivity of the nitrogen-doped TiO 2was due to the narrowing of the band gap by mixing the N 2p and O 2p states.We have previously reported 15that nitrogen-doped TiO 2powders irradiated with Vis light photo-catalytically decomposed CH 3CHO and 2-propanol into CO 2with different product distributions than irradiating with UV light.These experimental results suggested that the isolated N 2p narrow band that forms above the O 2p valence band was responsible for the visible light response and in the nitrogen-doped TiO 2the oxygen sites were substituted by nitrogen.In the present paper,the band structure of the TiO 2-x N x with small values of x (<0.02)was experimentally investigated by comparing the quantum efficiency of decomposing gaseous 2-propanol (IPA)under the same absorbed photon number of Vis or UV light.2.Experimental SectionTiO 2-x N x powders were prepared by annealing anatase TiO 2powder (ST01,Ishihara Sangyo Kaisha Ltd.)under NH 3flow at 550,575,and 600°C for 3h.TiO 2powder (ST01)was also annealed under an airflow at 550°C for 3h as a reference sample.An X-ray diffractometer (XRD),X-ray photoemission spec-troscopy (XPS),and UV -Vis light absorbance spectroscopy using the diffuse reflection method characterized the samples.The N 1s and O 1s core levels on the surface were measured by XPS in order to investigate the initial states of the NH 3and air annealed powders.According to Saha et al.,16the peak at 396eV is derived from Ti -N bonds.Therefore,the x values (nitrogen concentrations)were estimated by comparing the product of the 396eV peak area multiplied by the nitrogen sensitive factor to the product of the 531eV peak area (O 1s,Ti -O bonds)multiplied by the oxygen sensitive factor.*To whom correspondence should be addressed.Telephone:+81-3-5452-5080.Fax:+81-3-5452-5083.E-mail:kazuhito@fchem.chem.t.u-tokyo.ac.jp.5483J.Phys.Chem.B 2003,107,5483-548610.1021/jp030133h CCC:$25.00©2003American Chemical SocietyPublished on Web 05/17/2003The photocatalytic oxidation activities were evaluated by the decomposition of gaseous IPA when irradiating with Vis(400-530nm)or UV(300-400nm)light.The Vis and UV lights were obtained by a Xe lamp(Hayashi Tokei,Luminar Ace210) with glass filters(Toshiba Glass,B-47,L-42and C-40C)and by a black fluorescent light bulb(Toshiba Lighting&Technol-ogy)with a glass filter(Toshiba Glass,UV-D36B),respectively. Except when TiO2was irradiated with Vis light,the Vis or UV light intensity was calibrated for all the other sample powders to absorb1.4×1014quanta‚cm-2‚s-1,as shown in Table1. The irradiation area was approximately4.9cm2.A300mg sample of either TiO2-x N x or TiO2powders was uniformly spread over the irradiation area in a quartz-made vessel,which had a volume of500mL.About300ppm of the reactant gaswas injected.The samples were kept in the dark once the gas concentration remained constant,which implied that the IPA gas finished absorbing onto the powder surface.The component gas concentrations were measured using a gas chromatograph (Shimadzu model GC-8A).3.Results3.1.Sample Characterization.The powders annealed under NH3flow at550,575,and600°C were pale yellow,yellow, and dark green,respectively.The XRD patterns indicated that all the powders were a homogeneous anatase(TiO2)phase because only the peaks from the anatase phase and not TiN were observed.The powder annealed under airflow was white and identified as the homogeneous anatase phase.Figure1shows the N1s XPS spectra of TiO2-x N x and TiO2 powders.Since peaks at396eV,which have previously been found to result from Ti-N bonds,16are observed for the powders annealed under NH3,it was determined that the oxygen sites were substituted by nitrogen atoms.Since the XRD did not indicate the formation of TiN bonds,it was determined that O-Ti-N bonds formed.15Therefore,these powders were described as TiO2-x N x.In contrast,the air annealed samples did not display a peak at396eV and is TiO2.The peak around 400eV is the chemisorbed N2molecules,which absorbs onto the surface.16-19The estimated x values are listed in Table2. Figure2a shows the optical absorbance spectra obtained by the diffuse reflection of the TiO2-x N x and TiO2.Noticeable shifts of the absorption shoulders into the Vis light region were observed for TiO2-x N x.For x)0.019,the increase in absor-bance at wavelengths greater than550nm corresponds to Ti3+10 since NH3decomposes into N2and H2at around550°C20and H2is a reducing gas.TiO2is an indirect gap semiconductor,21 and the band gaps of the TiO2-x N xfrom the tangent lines in the plots ofKubelka-Munk functions against thein Figure2b.The tangent lines,which are extrapolated to R1/2)0,indicate the band gaps of the TiO2-xN x and TiO2are Eg)3.2eV,as shown in Figure2b.3.2.Photocatalytic Activity.Figure3shows the changes ofIPA,acetone,and CO2concentrations as a function of time inthe presence of TiO1.995N0.005powders while irradiating withVis light(0.645mW‚cm-2).Both acetone and CO2wereproduced when irradiating with Vis light,but the acetone furtherdecomposed into CO2.It was concluded that irradiating with TABLE1:Vis and UV Lights Intensity ax inTiO2-x N xVis light/(mW‚cm-2)UV light/(mW‚cm-2)x inTiO2-x N xVis light/(mW‚cm-2)UV light/(mW‚cm-2)00.645*0.1020.0110.3040.1020.00500.6450.1100.0190.1020.095a Absorbed photon number)1.0×1014quanta/(cm2‚s)(for the valuemarked with an asterisk,0quanta/(cm2‚s)).Figure1.N1s XPS spectra of TiO2-x N x and TiO2powders.Figure2.(a,upper)Optical absorbance spectra of TiO2-x N x and TiO2powders.(b,lower)Plots of the square root of the Kubelka-Munkfunction against the photon energy of TiO2-x N x and TiO2powders.Figure3.Changes of IPA,acetone,and CO2concentrations as afunction of time in the presence of TiO1.995N0.005powders under Vislight irradiation(0.645mW‚cm-2).TABLE2:x Values in TiO2-x N xannealingconditionx inTiO2-x N xannealingconditionx inTiO2-x N xair550°C0NH3575°C0.011NH3550°C0.0050NH3600°C0.019 5484J.Phys.Chem.B,Vol.107,No.23,2003Irie et al.Vis light in the presence of the photocatalyst completely decomposed the injected IPA because the evolved CO 2con-centration was 900ppm,which was three times the initial IPA concentration.Similar experiments were conducted with the other photo-catalysts.Figure 4shows the CO 2concentration changes for the TiO 2-x N x (x )0,0.0050,0.011,0.019)powders under Vis or UV irradiation,as listed in Table 1.As shown in Figure 4,irradiating TiO 2(TiO 2-x N x ,x )0)with Vis light did not generate CO 2as TiO 2is not Vis light sensitive.CO 2,however,was evolved from all the other sample powders by irradiating with Vis light.The CO 2concentration,900ppm,indicated that irradiating with Vis light in the presence of the photocatalysts completely decomposed IPA.23Irradiating the TiO 2-x N x (x )0.0050,0.011,0.019)powders with Vis light initially caused a lower CO 2generation rate.As the x values increased,a longer exposure to Vis light was required to decompose IPA.Irradiating with either UV or Vis light in the presence of TiO 2-x N x (x )0.0050,0.011,0.019)powders completely decomposed IPA.Figure 5shows the x value dependence on the quantum efficiency.Assuming that C 3H 8O +5H 2O +18h +f 3CO 2+18H +,the quantum efficiency (QY)was calculated 24using the following the equation,QY )(initial CO 2generation rate)/(calculated CO 2generation rate).The absorbed photon numbers were equivalent,1.4×1014quanta ‚cm -2‚s -1,for all the samples.The calculated CO 2generation rate was determined to be 1.9×10-10mol ‚s -1.The initial CO 2generation rate was calculated using the conventional least-squares method and the data in Figure 4.When irradiating with either Vis or UV light,the QY values decreased as x increased.The reason will be discussed later.4.DiscussionIrradiating TiO 2-x N x with UV light resulted in a higher QY value than irradiating with Vis light.This trend is plausible because the TiO 2oxygen lattice sites substituted by nitrogen atoms form an isolated narrow band above the valence band.Irradiating with UV light excites electrons in both the valence band and the narrow band,but irradiating with Vis light only excites electrons in the narrow band.If substituting nitrogenfor the oxygen sites in TiO 2narrows the band gap,then the QY values would be the same whether irradiating with Vis or UV light.The observations and Figure 2b do not support the narrowing of the band gap but are consistent with an isolated narrow band above the valence band,which produces the Vis light sensitive photocatalyst.Asahi et al .13calculated the band structure of TiO 2-x N x ,where x )0.25and 0.12,i.e.,when 12.5and 6at.%of the oxygen sites were substituted by nitrogen,and reported that mixing the N 2p and O 2p states narrowed the band gap.In our studies the x value in the TiO 2-x N x powders was much lower and at most was 0.02(1at.%).It is plausible that the band structure of the TiO 2-x N x with lower values of x (<0.02)should differ from higher x values (>0.12).The QY values of the TiO 2-x N x (x )0.0050,0.011,0.019)powders when irradiating with Vis light decreased as the x values increased.When annealing TiO 2powder under an NH 3atmosphere,the oxygen sites were partially replaced with nitrogen atoms while TiO 2was simultaneously reduced.20These changes caused an increase in oxygen vacancy and the amount of Ti 3+.The oxygen vacancy state in anatase TiO 2is below the lower end of the conduction band at 0.75-1.18eV 7and acts as a recombination center for holes and electrons.25As the NH 3annealing temperature was increased (with increasing x values),the more TiO 2reduction was reduced and the greater the number of oxygen vacancies,which explains why the QY values were lower as x increased.In fact,TiO 1.981N 0.019powders had an absorbance greater than 550nm,which corresponds to Ti 3+10(Figure 2a).The same trend was also observed when TiO 2-x N x (x )0.0050,0.011,0.019)powders were irradiated with UV light because the oxygen vacancies acted as recombination centers for holes and electrons.5.ConclusionTiO 2-x N x (x )0,0.0050,0.011,0.019)powders were prepared by annealing under an NH 3flow at 550,575,and 600°C.XRD and XPS confirmed that the prepared powders had nitrogen substituted at some of the oxygen sites in TiO 2,which formed a narrow N 2p band above the valence band.It was determined that the narrow N 2p band was responsible for the visible light sensitivity by considering the QY values,which were calculated by the CO 2generation rate by decomposing IPA in Vis or UV light at a constant photon number (1.4×1014quanta ‚cm -2‚s -1).When irradiating with Vis light,the QY values decreased as x increased.This is because the oxygen vacancies,which promote the recombination of holes and electrons,increased with x .When irradiating with UV light,the QY values decreased as the nitrogen concentration increased,indicating that the doping sites could serve as recombination centers,too.Acknowledgment.This work was supported by a Grant-in-Aid for Scientific Research on Priority Areas (Grant 417)from the Ministry of Education,Culture,Sports,Science and Technology (MEXT)of the Japanese Government.References and Notes(1)Heller,A.Acc.Chem.Res.1995,28,141.(2)Linsebigler,A.L.;Lu,G.Q.;Yates J.T.Chem.Re V .1995,95,735.(3)Fujishima,A.;Hashimoto,K.;Watanabe,T.TiO 2Photocatalysis,Fundamentals and Applications ;BKC,Inc.:Tokyo,1999.(4)Borgarello,E.;Kiwi,J.;Gratzel,M.;Pelizzetti,E.;Visca,M.J.Am.Chem.Soc.1982,104,2996.(5)Yamashita,H.;Ichihashi,Y.;Takeuchi,M.;Kishiguchi,S.;Anpo,M.J.Synchrotron Radiat.1999,6,451.(6)Anpo,M.;Takeuchi,M.Int.J.Photoenergy 2001,3,1.Figure 4.CO 2concentration changes for the TiO 2-x N x (x )0,0.0050,0.011,0.019)powders under Vis or UV lightirradiation.Figure 5.x value dependence of the quantum efficiency.Photocatalytic Activity of TiO 2-x N x Powders J.Phys.Chem.B,Vol.107,No.23,20035485(7)Nakamura,I.;Negishi,N.;Kutsuna,S.;Ihara,T.;Sugihara,S.; Takeuchi,K.J.Mol.Catal.A:Chem.2000,161,205.(8)Ando,K.;Ando,M.;Fujii,M.Photocatalysis2001,6,20.(9)Sato,S.Chem.Phys.Lett.1986,123,126.(10)Noda,H.;Oikawa,K.;Ogata,T.;Matsuki,K.;Kamata,H.Chem. Soc.Jpn.1986,8,1084.(11)Sakatani,Y.;Koike,H.Japan Patent,P2001-72419A,2001.(12)Ihara,T.;Ando,M.;Sugihara,S.Photocatalysis2001,5,19.(13)Asahi,R.;Morikawa,T.;Ohwaki,T.;Aoki,K.;Taga,Y.Science 2001,293,269.(14)Morikawa,T.;Asahi,R.;Ohwaki,T.;Taga,Y.PCT WO01/10553 A1,2001.(15)Irie,H.;Kako,T.;Nakajima,A.;Watanabe,T.;Hashimoto,K.J. Photochem.Photobiol.,A,submitted for publication.(16)Saha,N.C.;Tompkins,H.G.J.Appl.Phys.1992,72,3072.(17)Fuggle,J.C.;Umbach,E.;Menzel,D.;Wandelt,K.;Brundle,C. R.Solid State Commun.1978,27,65.(18)Wu,H.Z.;Chou,T.C.;Mishra,A.;Anderson,D.R.;Lampert,J. K.;Gujrathi,S.C.Thin Solid Films1990,191,55.(19)Shinn N.D.;Tsang,K.L.J.Vac.Sci.Technol.,A1991,9(3), 1558.(20)Shin,C.H.;Bugli,G.;Djega-Mariadassou,G.;J.Solid State Chem. 1991,95,145.(21)Koffyberg,F.P.;Dwight,K.;Wold,A.Solid State Commun.1979, 30,433.(22)Kim,Y.I.;Atherton,S.J.;Brigham,E.S.;Mallouk,T.E.J.Phys. Chem.1993,97,11802.(23)The CO2concentration in the presence of TiO1.981N0.019powder is around300ppm in Figure4,but the complete IPA decomposition and the formation of900ppm of CO2was confirmed.(24)Hashimoto,K.;Fujishima,A.TiO2Photocatalysis:Its Application to Self-Cleaning,Anti-bacterial and Air Purifying Materials;1998.(25)The oxygen vacancy state(Ti3d impurity level)is not visible light sensitive.In fact,hydrogen-reduced TiO2(TiO2-x)powders,which have gray color,are not visible light sensitive.The N2p narrow band exists much deeper than the Ti3d donor level,and the N2p level has a lot more oxidation power than the oxygen vacancy level.Therefore,the origin of visible light sensitivity in TiO2-x N x is the N2p narrow band level and not the oxygen vacancy level.5486J.Phys.Chem.B,Vol.107,No.23,2003Irie et al.。