Effect of machining parameters and heat treatment on the
机械加工刀具几何形状论文中英文资料对照外文翻译
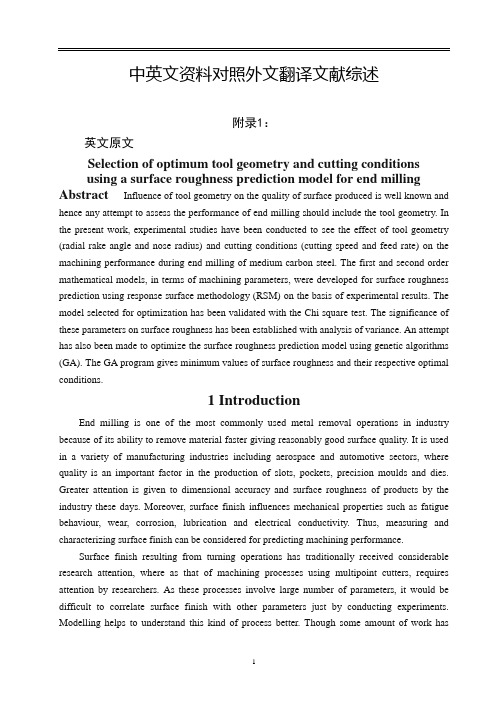
中英文资料对照外文翻译文献综述附录1:英文原文Selection of optimum tool geometry and cutting conditionsusing a surface roughness prediction model for end milling Abstract Influence of tool geometry on the quality of surface produced is well known and hence any attempt to assess the performance of end milling should include the tool geometry. In the present work, experimental studies have been conducted to see the effect of tool geometry (radial rake angle and nose radius) and cutting conditions (cutting speed and feed rate) on the machining performance during end milling of medium carbon steel. The first and second order mathematical models, in terms of machining parameters, were developed for surface roughness prediction using response surface methodology (RSM) on the basis of experimental results. The model selected for optimization has been validated with the Chi square test. The significance of these parameters on surface roughness has been established with analysis of variance. An attempt has also been made to optimize the surface roughness prediction model using genetic algorithms (GA). The GA program gives minimum values of surface roughness and their respective optimal conditions.1 IntroductionEnd milling is one of the most commonly used metal removal operations in industry because of its ability to remove material faster giving reasonably good surface quality. It is used in a variety of manufacturing industries including aerospace and automotive sectors, where quality is an important factor in the production of slots, pockets, precision moulds and dies. Greater attention is given to dimensional accuracy and surface roughness of products by the industry these days. Moreover, surface finish influences mechanical properties such as fatigue behaviour, wear, corrosion, lubrication and electrical conductivity. Thus, measuring and characterizing surface finish can be considered for predicting machining performance.Surface finish resulting from turning operations has traditionally received considerable research attention, where as that of machining processes using multipoint cutters, requires attention by researchers. As these processes involve large number of parameters, it would be difficult to correlate surface finish with other parameters just by conducting experiments. Modelling helps to understand this kind of process better. Though some amount of work hasbeen carried out to develop surface finish prediction models in the past, the effect of tool geometry has received little attention. However, the radial rake angle has a major affect on the power consumption apart from tangential and radial forces. It also influences chip curling and modifies chip flow direction. In addition to this, researchers [1] have also observed that the nose radius plays a significant role in affecting the surface finish. Therefore the development of a good model should involve the radial rake angle and nose radius along with other relevant factors.Establishment of efficient machining parameters has been a problem that has confronted manufacturing industries for nearly a century, and is still the subject of many studies. Obtaining optimum machining parameters is of great concern in manufacturing industries, where the economy of machining operation plays a key role in the competitive market. In material removal processes, an improper selection of cutting conditions cause surfaces with high roughness and dimensional errors, and it is even possible that dynamic phenomena due to auto excited vibrations may set in [2]. In view of the significant role that the milling operation plays in today’s manufacturing world, there is a need to optimize the machining parameters for this operation. So, an effort has been made in this paper to see the influence of tool geometry(radial rake angle and nose radius) and cutting conditions(cutting speed and feed rate) on the surface finish produced during end milling of medium carbon steel. The experimental results of this work will be used to relate cutting speed, feed rate, radial rake angle and nose radius with the machining response i.e. surface roughness by modelling. The mathematical models thus developed are further utilized to find the optimum process parameters using genetic algorithms.2 ReviewProcess modelling and optimization are two important issues in manufacturing. The manufacturing processes are characterized by a multiplicity of dynamically interacting process variables. Surface finish has been an important factor of machining in predicting performance of any machining operation. In order to develop and optimize a surface roughness model, it is essential to understand the current status of work in this area.Davis et al. [3] have investigated the cutting performance of five end mills having various helix angles. Cutting tests were performed on aluminium alloy L 65 for three milling processes (face, slot and side), in which cutting force, surface roughness and concavity of a machined plane surface were measured. The central composite design was used to decide on the number of experiments to be conducted. The cutting performance of the end mills was assessed using variance analysis. The affects of spindle speed, depth of cut and feed rate on the cutting force and surface roughness were studied. The investigation showed that end mills with left hand helixangles are generally less cost effective than those with right hand helix angles. There is no significant difference between up milling and down milling with regard tothe cutting force, although the difference between them regarding the surface roughness was large. Bayoumi et al.[4] have studied the affect of the tool rotation angle, feed rate and cutting speed on the mechanistic process parameters (pressure, friction parameter) for end milling operation with three commercially available workpiece materials, 11 L 17 free machining steel, 62- 35-3 free machining brass and 2024 aluminium using a single fluted HSS milling cutter. It has been found that pressure and friction act on the chip – tool interface decrease with the increase of feed rate and with the decrease of the flow angle, while the cutting speed has a negligible effect on some of the material dependent parameters. Process parameters are summarized into empirical equations as functions of feed rate and tool rotation angle for each work material. However, researchers have not taken into account the effects of cutting conditions and tool geometry simultaneously; besides these studies have not considered the optimization of the cutting process.As end milling is a process which involves a large number f parameters, combined influence of the significant parameters an only be obtained by modelling. Mansour and Abdallaet al. [5] have developed a surface roughness model for the end milling of EN32M (a semi-free cutting carbon case hardening steel with improved merchantability). The mathematical model has been developed in terms of cutting speed, feed rate and axial depth of cut. The affect of these parameters on the surface roughness has been carried out using response surface methodology (RSM). A first order equation covering the speed range of 30–35 m/min and a second order equation covering the speed range of 24–38 m/min were developed under dry machining conditions. Alauddin et al. [6] developed a surface roughness model using RSM for the end milling of 190 BHN steel. First and second order models were constructed along with contour graphs for the selection of the proper combination of cutting speed and feed to increase the metal removal rate without sacrificing surface quality. Hasmi et al. [7] also used the RSM model for assessing the influence of the workpiece material on the surface roughness of the machined surfaces. The model was developed for milling operation by conducting experiments on steel specimens. The expression shows, the relationship between the surface roughness and the various parameters; namely, the cutting speed, feed and depth of cut. The above models have not considered the affect of tool geometry on surface roughness.Since the turn of the century quite a large number of attempts have been made to find optimum values of machining parameters. Uses of many methods have been reported in the literature to solve optimization problems for machining parameters. Jain and Jain [8] have used neural networks for modeling and optimizing the machining conditions. The results have been validated by comparing the optimized machining conditions obtained using genetic algorithms.Suresh et al. [9] have developed a surface roughness prediction model for turning mild steel using a response surface methodology to produce the factor affects of the individual process parameters. They have also optimized the turning process using the surface roughness prediction model as the objective function. Considering the above, an attempt has been made in this work to develop a surface roughness model with tool geometry and cutting conditions on the basis of experimental results and then optimize it for the selection of these parameters within the given constraints in the end milling operation.3 MethodologyIn this work, mathematical models have been developed using experimental results with the help of response surface methodolog y. The purpose of developing mathematical models relating the machining responses and their factors is to facilitate the optimization of the machining process. This mathematical model has been used as an objective function and the optimization was carried out with the help of genetic algorithms.3.1 Mathematical formulationResponse surface methodology(RSM) is a combination of mathematical and statistical techniques useful for modelling and analyzing the problems in which several independent variables influence a dependent variable or response. The mathematical models commonly used are represented by:where Y is the machining response, ϕ is the response function and S, f , α, r are milling variables and ∈is the error which is normally distributed about the observed response Y with zero mean.The relationship between surface roughness and other independent variables can be represented as follows,where C is a constant and a, b, c and d are exponents.To facilitate the determination of constants and exponents, this mathematical model will have to be linearized by performing a logarithmic transformation as follows:The constants and exponents C, a, b, c and d can be determined by the method of least squares. The first order linear model, developed from the above functional relationship using least squares method, can be represented as follows:where Y1 is the estimated response based on the first-order equation, Y is the measured surface roughness on a logarithmic scale, x0 = 1 (dummy variable), x1, x2, x3 and x4 are logarithmic transformations of cutting speed, feed rate, radial rake angle and nose radius respectively, ∈is the experimental error and b values are the estimates of corresponding parameters.The general second order polynomial response is as given below:where Y2 is the estimated response based on the second order equation. The parameters, i.e. b0, b1, b2, b3, b4, b12, b23, b14, etc. are to be estimated by the method of least squares. Validity of the selected model used for optimizing the process parameters has been tested with the help of statistical tests, such as F-test, chi square test, etc. [10].3.2 Optimization using genetic algorithmsMost of the researchers have used traditional optimization techniques for solving machining problems. The traditional methods of optimization and search do not fare well over a broad spectrum of problem domains. Traditional techniques are not efficient when the practical search space is too large. These algorithms are not robust. They are inclined to obtain a local optimal solution. Numerous constraints and number of passes make the machining optimization problem more complicated. So, it was decided to employ genetic algorithms as an optimization technique. GA come under the class of non-traditional search and optimization techniques. GA are different from traditional optimization techniques in the following ways:1.GA work with a coding of the parameter set, not the parameter themselves.2.GA search from a population of points and not a single point.3.GA use information of fitness function, not derivatives or other auxiliary knowledge.4.GA use probabilistic transition rules not deterministic rules.5.It is very likely that the expected GA solution will be the global solution.Genetic algorithms (GA) form a class of adaptive heuristics based on principles derived from the dynamics of natural population genetics. The searching process simulates the natural evaluation of biological creatures and turns out to be an intelligent exploitation of a random search. The mechanics of a GA is simple, involving copying of binary strings. Simplicity of operation and computational efficiency are the two main attractions of the genetic algorithmic approach. The computations are carried out in three stages to get a result in one generation or iteration. The three stages are reproduction, crossover and mutation.In order to use GA to solve any problem, the variable is typically encoded into a string (binary coding) or chromosome structure which represents a possible solution to the given problem. GA begin with a population of strings (individuals) created at random. The fitness of each individual string is evaluated with respect to the given objective function. Then this initial population is operated on by three main operators – reproduction cross over and mutation– to create, hopefully, a better population. Highly fit individuals or solutions are given the opportunity to reproduce by exchanging pieces of their genetic information, in the crossover procedure, with other highly fit individuals. This produces new “offspring” solutions, whichshare some characteristics taken from both the parents. Mutation is often applied after crossover by altering some genes (i.e. bits) in the offspring. The offspring can either replace the whole population (generational approach) or replace less fit individuals (steady state approach). This new population is further evaluated and tested for some termination criteria. The reproduction-cross over mutation- evaluation cycle is repeated until the termination criteria are met.中文翻译选择最佳工具,几何形状和切削条件利用表面粗糙度预测模型端铣摘要:刀具几何形状对工件表面质量产生的影响是人所共知的,因此,任何成型面端铣设计应包括刀具的几何形状。
35CrMo钢钻头锻造开裂原因分析
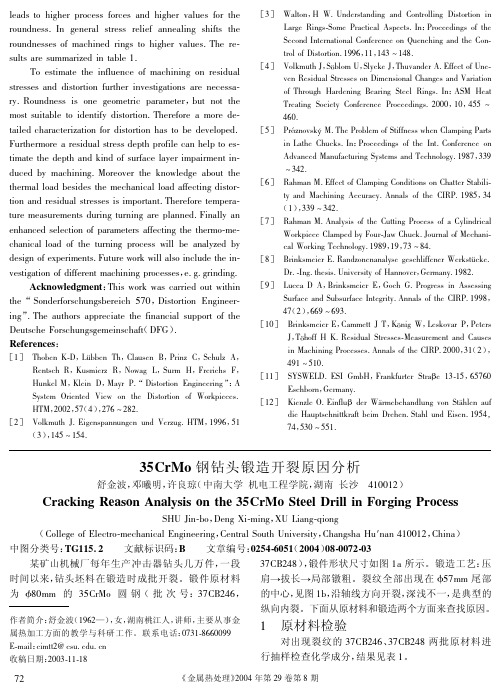
35crmo 钢钻头锻造开裂原因分析
舒金波, 邓曦明, 许良琼 ( 中南大学 机电工程学院, 湖南 长沙 410012 )
cracking Reason Analysis on the 35crmo Steel Drill in Forging Process
SHU Jin-bo, Deng Xi-ming, XU Liang-giong ( CoIIege of EIectro-mechanicaI Engineering, CentraI South University, Changsha HuInan 410012 , China) 中图分类号: TG115. 2 文献标识码: B 文章编号: 0254-6051 ( 2004 ) 08-0072-03 37CB248 ) , 锻件形状尺寸如图 1a 所示。锻造工艺: 压 肩、拔长、局部镦粗。裂纹全部出现在 #57mm 尾部 的中心, 见图 1b, 沿轴线方向开裂, 深浅不一, 是典型的 纵向内裂。下面从原材料和锻造两个方面来查找原因。
( 2 ) 不均匀变形导致锻坯中心部位出现附加拉 应力 观察锻坯产生裂纹的部位和形状特征, 其为圆 形坯料在平砧上拔长时常见的一种锻造缺陷。用平砧 拔长圆断面毛坯时, 若压下量较小, 则砧子与金属坯料 的接触面较窄长, 砧子与金属接触的开始瞬间是一条
作者简介: 舒金波 ( 1962 —) , 女, 湖南桃江人, 讲师, 主要从事金 属热加工方面的教学与科研工作。联系电话: 0731-8660099 E-maiI: cimtt2@ csu. edu. cn 收稿日期: 2003-11-18
某矿山机械厂每年生产冲击器钻头几万件, 一段 时间以来, 钻头坯料在锻造时成批开裂。锻件原材料 为 !80mm 的 35CrMo 圆 钢( 批 次 号:37CB246 ,Байду номын сангаас
数据车床加工精度(Machining accuracy of data lathe)
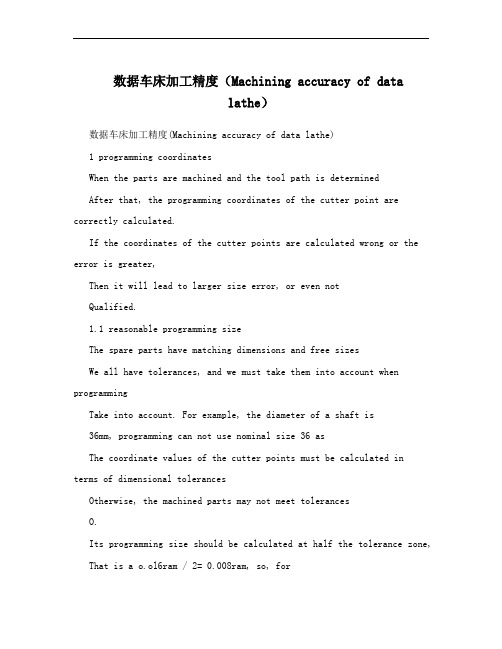
数据车床加工精度(Machining accuracy of datalathe)数据车床加工精度(Machining accuracy of data lathe)1 programming coordinatesWhen the parts are machined and the tool path is determinedAfter that, the programming coordinates of the cutter point are correctly calculated.If the coordinates of the cutter points are calculated wrong or the error is greater,Then it will lead to larger size error, or even notQualified.1.1 reasonable programming sizeThe spare parts have matching dimensions and free sizesWe all have tolerances, and we must take them into account when programmingTake into account. For example, the diameter of a shaft is36mm, programming can not use nominal size 36 asThe coordinate values of the cutter points must be calculated interms of dimensional tolerancesOtherwise, the machined parts may not meet tolerancesO.Its programming size should be calculated at half the tolerance zone, That is a o.ol6ram / 2= 0.008ram, so, forCheng Chicun: 35.992ram.Also, for example, 5 0h 7, check match tolerances, tolerancesIs 50ram, the value of a 0.025mm / tolerance:2= 1, 0, l, 25mm, programming size: 49.988mm.1.2 calculation of inside diameter programming dimension when machining threadWhen machining threads, there is a squeeze out of the toolThe outer diameter of the outer thread becomes larger while the inner screw is pressedThe phenomenon that the inner diameter of the thread becomes smaller affects the fit of the threadIn order to consider this size change in programming.Calculation of 1.2.1 major diameter of external threadIn general, the programming dimensions of the outer thread diameter are:The nominal diameter of the outer thread is subtracted from the 0.1 pitch. Such as:Processing M30, X, 2ram external thread, turning outside the circle The diameter should be 30 - 0.1 X 2:29.8ram asProgramming size.Calculation of 1.2.2 thread diameterIn general, the bore diameter of the inner thread is greater than that of the inner threadThe nominal diameter of a pitch value. For example, turning M 40A programming rule for the bore diameter of an internal thread of X 2mmInch should be: 38mm.2 Effect of tool selectionAccording to the materials, machining parts, performance andShape of surface, choose the type and grade of cutters reasonably And geometric parameters of cutting tools. Such as the rake angle and the rake angle of the toolAnd the trimming blade can improve the surface processing quality of the partsA great role.When the curved surface of the machined part is curvedWhen the requirements of shape accuracy and surface roughness are higher, they are neededThe arc turning tool for machining.3 Effect of cutting parameters selectionCutting parameters including cutting depth, feed speed andThe cutting speed, also called the three elements of cutting. These three parametersThe choice of the right, will directly affect the processing of partsAccuracy and surface roughness.The stiffness of the process system and the power of the latheduring roughingUnder the allowable conditions, in order to improve the processing efficiency, the general electionChoose the larger cutting depth, cutting depth, cutting forceLarge, the tool and parts prone to deformation, will affectMachining accuracy.The cutting depth is determined, according to the depth of selected.Optional feed rateAccording to the depth of the selected amount, feeding speed.Pieces can be calculated by empirical formula, or according toactual productionThe experience of table look-up selection cutting speed.When the precision requirements of parts are higher, we should consider leaving themMake proper allowance for the car, and determine the back of the car according to the margin of the carEngagement, fine car should choose smaller feed speed andHigher cutting speed.4 the influence of test cutting tool settingThe precision of tool cutting and the speed of tool setting are directly affectedSound to the quality and efficiency of the process. For economical CNC machinesBed, mostly manual test cutting tool. Guang you GuangNumerical GSK9 80T lathe CNC system as an example to illustrate the rightInfluence of cutting process on machining accuracy.When machining parts are entered into the lathe, and thenWhen the test is correct, the test can be carried outThe knives and parts have been machined, and the steps of cutting and cutting are as follows:(1) mechanical return to zero, U and W relative coordinate value cleared;(2) cutting the surface of a part with a reference knife is usually used90. The excircle tool is clamped at 0 L number and is used asthe benchmarkKnife. When the programming coordinate origin is located at theright end of the part, the cross section is formedWhen the heart is in hand, try to cut the end face manually and retract the knife along the X axis, ZThe shaft cannot move. Press the knife key, turn the cursor after pageMove to "knife number plus l00", that is, on the line l0l, press w, 0, IN key. Then, 0l knife, Z axis of the knife will be biased into the valueCNC system.Try to cut the outer circle and retract along the Z axis. The X axis is squareBe unable to move. Stop spindle rotation and measure the cutting positionThe diameter of the part, and then press the X key to enter the measuredDiameter value (must enter decimal point), and then press the IN key. be0l knife, X axis of the knife will enter the bias value.If the diameter of the measurement is inaccurate, then the inputThere is an error in the offset of the cutter, and the machined part must also be offsetBut I can't guarantee the size. So the No. 0 l knife sets the tool The key lies in the accuracy of part diameter measurement.72 science and Technology Innovation Herald Science, and, Technology, Innovation, HeraldIndustrial Technology(3) the presetting step of the non reference cutter and thereference cutter are basicSame. The difference is that the knife point of the non reference knife isClose the part surface instead of cutting the part.The key point of non reference cutter is tool location and zeroThe closeness of a piece is not cut, but cutSample, the input knife bias will be correct, the processing of zero To meet the size requirements.(4) the direction of the Z axis of the screw cutter is limited when the tool is setThe amount of error does not affect the machining accuracy of the parts, while the X axis sideThere is no error in direction.5 conclusionsThe above analysis of the impact of CNC lathe processing fine magicA few factors, which can not be ignored, or will be depositedThe small error is a big error so that the machined part is not upto standard.Students in the training process, the use of the same car cheap t ah Same processing program, cutting tool. The machining error is large Have small. The main reason is that the cutting tool is inaccurate The diameter of the reference cutter is not accurate enough tomeasure the accuracy of the TQuasi knife, knife was not completely attached to the workpiece, seamGap or cut into the surface of the part; followed by data inputOr do not follow the steps of cutting the tool.This leads directly to the size of the processing errorIn addition, tool wear, workpiece blank stiffness are alsoIs the cause of machining errors. OneReference[l] Chen Yunqing. CNC lathe programming and skills training. North Beijing: Chemical Industry Press, 2006, 6.[2] Yan Chuhong.NC machining technology and programming BeijingAcademic industry press, 2004, 3.[3] Xie Xiaohong. NC turning programming and processing technology. NorthBeijing: Publishing House of electronics industry, 2005, 7。
形状记忆合金的材料参数和体积分数对大块金属玻璃增韧的影响
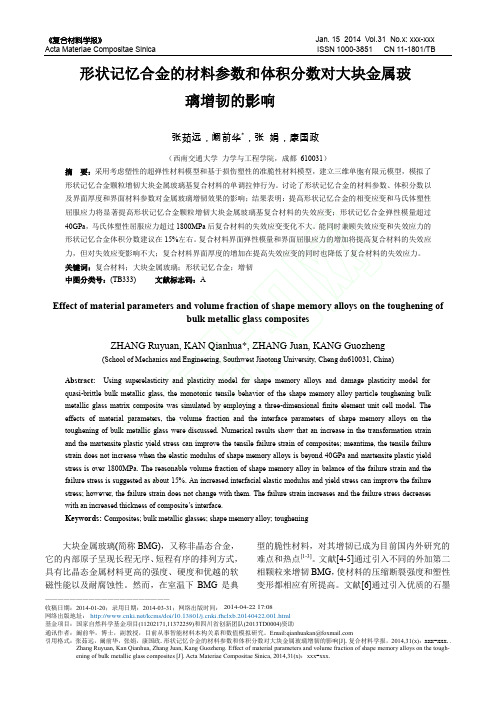
《复合材料学报》 Jan. 15 2014 Vol.31 No.x: xxx-xxx Acta Materiae Compositae Sinica ISSN 1000-3851 CN 11-1801/TB————————————————————收稿日期:2014-01-20;录用日期:2014-03-31;网络出版时间: 网络出版地址: 基金项目:国家自然科学基金项目(11202171,11372259)和四川省创新团队(2013TD0004)资助通讯作者:阚前华,博士,副教授,目前从事智能材料本构关系和数值模拟研究。
Email:**********************引用格式:张茹远,阚前华,张娟,康国政.形状记忆合金的材料参数和体积分数对大块金属玻璃增韧的影响[J].复合材料学报,2014,31(x):xxx-xxx.. Zhang Ruyuan, Kan Qianhua, Zhang Juan, Kang Guozheng. Effect of material parameters and volume fraction of shape memory alloys on the tough-ening of bulk metallic glass composites [J]. Acta Materiae Compositae Sinica, 2014,31(x):xxx -xxx.形状记忆合金的材料参数和体积分数对大块金属玻璃增韧的影响张茹远,阚前华*,张 娟,康国政(西南交通大学 力学与工程学院,成都 610031)摘 要:采用考虑塑性的超弹性材料模型和基于损伤塑性的准脆性材料模型,建立三维单胞有限元模型,模拟了形状记忆合金颗粒增韧大块金属玻璃基复合材料的单调拉伸行为。
讨论了形状记忆合金的材料参数、体积分数以及界面厚度和界面材料参数对金属玻璃增韧效果的影响;结果表明:提高形状记忆合金的相变应变和马氏体塑性屈服应力将显著提高形状记忆合金颗粒增韧大块金属玻璃基复合材料的失效应变;形状记忆合金弹性模量超过40GPa ,马氏体塑性屈服应力超过1800MPa 后复合材料的失效应变变化不大。
Effect of the cutting parameters impact on tool life in duplex stainless steel turning process

See discussions, stats, and author profiles for this publication at: https:///publication/256129621Effect of the cutting parameters impact on tool life in duplex stainless steel turning processArticle in Tehnicki Vjesnik · August 2013Impact Factor: 0.58CITATIONS 19READS1,4003 authors:Grzegorz KrolczykOpole University of Technology61 PUBLICATIONS 250 CITATIONSSEE PROFILEMaksymilian GajekOpole University of Technology8 PUBLICATIONS 74 CITATIONSSEE PROFILEStanislaw LegutkoPoznan University of Technology66 PUBLICATIONS 260 CITATIONSSEE PROFILEAll in-text references underlined in blue are linked to publications on ResearchGate,Available from: Grzegorz KrolczykG. Królczyk et al. Utjecaj parametara obrade na vijek alata u procesu tokarenja dupleks čelika otpornog na korozijuISSN 1330-3651 (Print), ISSN 1848-6339 (Online)UDC/UDK 620.178.1:[621.941.025:669.14] EFFECT OF THE CUTTING PARAMETERS IMPACT ON TOOL LIFE IN DUPLEX STAINLESS STEEL TURNING PROCESSGrzegorz Królczyk, Maksymilian Gajek, S tanisław LegutkoOriginal scientific paper The purpose of the study is to determine the coated carbide tool life and the tool point surface topography. The study included determining cutting conditions in the process of turning the DSS and designating the wear curve. In case of machining greater resistance to abrasive wear of the tools which were coated with Al2O3 has been demonstrated. Metallographic microscopy has been used for the microstructure of the surface layer analysis.Keywords: Duplex Stainless Steel, machining, tool life, turning, wearUtjecaj parametara obrade na vijek alata u procesu tokarenja dupleks čelika otpornog na korozijuIzvorni znanstveni članak Ova je analiza provedena kako bi se odredio vijek alata presvuće nog karbidom i topografija površine vrha alata. Analiza je uključila određivanje uvjeta rezanja u postupku tokarenja dupleks čelika otpornog na koroziju (DDS)i označavanje krivul je trošenja. Kod strojne obrade pokazala se veća otpornost na abrazivno trošenje onih alata koji su bili presvućeni Al2O3. Analiza mikrostrukture površinskog sloja je izvršena pomoću metalografskog mikroskopa.Ključne riječi: duple ks čelik otporan na koroziju, strojna obrada, tokarenje, trošenje, vijek alata1 IntroductionAccording to companies producing construction materials - duplex stainless steel is gaining importance, which is reflected in the wide range of these products available in the market. However, the manufacturing process, the machining in particular, poses considerable difficulties. One limitation of the efficiency of turning this type of steel is the wear of the tool point. The wearing process of the tool point, which is largely dependent on cutting parameters, is an important factor. The wear of the tool point leads to deterioration in quality of machined surface and, consequently, to lower efficiency and productivity. Machining DSS due to the characteristic two-phase microstructure is difficult and in order to overcome occurring problems, materials with high durability, reliability and efficiency should be used. In recent years, machinability of austenitic steels has been dealt with by researchers such as Paro, J. et al., Akasawa T. et al., Abou-El-Hossein K. A. et al., Charles J. et al., KosmačA., Cunat P. J. and Ciftci I. [1 ÷ 8], while machining of DSS has been described by Bouzid Sai W. and J. L. Lebrun [9]. Many production companies use coated carbide tools or high speed steel for processing of DSS. According to Gunn'a [10] low-alloyed DSS such as S32304 while being machined by tools from high speed steels behave in a manner similar to austenitic types such as 316 or 317. However, during the machining of coated carbide tools steel behaves in a manner similar to 317LN and 317LMN. Modern types of DSS are harder to machine than the types produced before this one. The reason for this is higher content of austenite phase and nitrogen. The increase in content of alloying elements such as nitrogen and molybdenum makes machinability of these steels less effective. The use of coated carbide tools for machining of DSS requires a deeper study of tool wear and associated wear mechanisms. The article focuses on basic research problems of tool wear of coated carbide with a layer of CVD-Ti (C,N)/Al2O3/TiN in turning DSS of ferritic-austenitic structure. The main purpose of this study was to determine the effect of cutting speed as a key process factor controlling tool life. Increasing cutting speed to a scope greatly exceeding conventional machining is now recognized as the primary direction of production capacity and efficiency growth as well as quality and accuracy improvement [11]. As the method of rational selection for DSS machining a static determined selective-multivariate uniform static - rotatable PS/S-P:λ program has been selected [12 ÷ 14]. The research program included an assessment of influence of cutting parameters impact onto tool life, rake face as well as flank wear in the process of turning. Tool wear data were used to determine characteristic wear curves.2 Experimental techniques2.1 Workpiece and cutting tool materialsMachined material was 1.4462 (DIN EN 10088-1) steel with a ferritic-austenitic structure containing about 50 % of austenite. The ultimate tensile strength R m = 700 MPa, Brinell hardness - 293±3 HB. The elemental composition of the machined material and technical details of the cutting tools are given in Tabs. 1 and 2 respectively. Cutting tool inserts of TNMG 160408 designation clamped in the tool shank of ISO-MTGNL 2020-16 type were employed.Table 1 Chemical composition of 1.4462 duplex stainless steelElementCmaxSimaxMnmaxPmaxSmax Cr Ni Mo NOthers%wt. 0,03 1,00 2,00 0,030 0,02021,023,04,506,502,503,500,100,22 - Based on the industry recommendations a range of cutting parameters T1: v c = 50 ÷ 150 m/min, f = 0,2 ÷ 0,4 mm/rev, a p= 1 ÷ 3 mm was selected. The experiments performed with the tool point T2 were comparative studies and that is why the cutting parameters were: v c =Effect of the cutting parameters impact on tool life in duplex stainless steel turning process G. Królczyk et al.50, 100 and 150 m/min, f = 0,2; 0,3 and 0,4 mm/rev, a p = 2 mm. The study was conducted within a production facility. The research program was carried out on a CNC lathe 400 CNC Famot Pleszew plc.Table 2 Cutting tool specificationToolSubstrate OthersT1 MM 2025Hardness: 1350 HV3 Grade: M25, P35Coatings: Ti(C,N)-(2 µm) (Top layer)Al 2O 3-(1,5 µm) (Middle layer)TiN-(2 µm) (Bottom layer)Coating technique: CVD T2 CTC 1135Grade: M35, P35Coatings: TiN-(2 µm) (Top layer) Ti(C,N)-(2 µm) Ti(N,B)-(2 µm) TiN-(2 µm) Ti(C,N)-(2 µm)Ti(C,N)-(2 µm) (Bottom layer)Coating technique: CVDTool geometry (TNMG 160408):l = 16,50 mm d = 9,52 mm s = 4,76 mm d 1 = 3,81 mm r = 0,8 mmTable 3 Coded indications of the study planNo. x 1x 2x 3v c / m/min f / mm/rev a p / mm1 −1 −1 −1 70 0,24 1,42 −1 −1 +1 70 0,24 2,63 −1 +1 −1 70 0,36 1,4 4 −1 +1 +1 70 0,36 2,65 +1 −1 −1 130 0,24 1,46 +1 −1 +1 130 0,24 2,67 +1 +1 −1 130 0,36 1,48 +1 +1 +1 130 0,36 2,69 −1,682 0 0 50 0,3 2 10 1,682 0 0 150 0,3 2 11 0 −1,682 0 100 0,2 2 12 0 1,682 0 100 0,4 2 13 0 0 −1,682 100 0,3 1 14 0 0 1,682 100 0,3 3 15 0 0 0 100 0,3 2 16 0 0 0 100 0,3 2 17 0 0 0 100 0,3 2 18 0 0 0 100 0,3 2 19 0 0 0 100 0,3 2 200 0 0 100 0,3 22.2 Tool life planThe required number of experimental points is N =23+ 6 + 6 =20 (Tab. 3). There are eight factorial experiments (3 factors on two levels, 23) with added 6 star points and centre point (average level) repeated 6 times to calculate the pure error [15]. For the purpose of the experiment a program that estimates parameters of the model second-order polynomial in the form y = (a 0 + a 1∙x 1 + a 2∙x 2 + a 3∙x 3)2 has been developed. The program was written in Matlab and it allows generating three-dimensional graphs and plots of one variable. The tests were performed on a CNC lathe, hence the test plan had been adjusted to the GE Fanuc Series 0 - T controlled machine program.2.3 Wear analysisAfter cutting attempts values of flank wear were measured with the use of an optical microscope.3 Results and discussion 3.1 Wear curvesThe examination of the process of the tool point wear particularly in industrial processes showed that the most common type of wear was the average and maximum wear bandwidth of abrasive wear on the major flank in zone B - respectively VB B (Fig. 1) and VB Bmax (Fig . 2). Therefore, the experiment adopted this kind of criterion. Tool-life curves were determined for the center parameters of a research program for the T1 tool point. As one may notice, the VB B curve (Fig. 1) is typical of the steel machining with the average cutting speed, with no special cooling or of little intense cooling. This may indicate a three discernible, typical periods of tool wear. While analysing the results for VB Bmax wear curve (Fig . 2), a greater value of wear can be noticed; this may indicate irregularly worn major flank.Figure 1 Tool wear VB B for coated carbide tools T1Figure 2 Tool wear VB Bmax for coated carbide tools T13.2 Tool lifeFig. 3 shows the tool life after machining DSS with T1 tool under dry cutting conditions. The results obtained by modelling on the basis of adopted program PS/DS-P:λ were presented as a three-dimensional plot and two plots of one variable in sequence showing the depth of cut and cutting speed for the parameters from the point of the centre. For a f = 0,3 mm/rev feed and cutting speed of v c = 100 m/min the tool life of the tool point takes the greatest value for the depth of cut a p = 1 mm and a p = 3 mm and amounts to T = 31 min and T = 23 min. The minimum value was observed for the depth of cut of a p = 2,3 mm at the tool life amounting to T = 20 min. For the f = 0,3G. Królczyk et al. Utjecaj parametara obrade na vijek alata u procesu tokarenja dupleks čelika otpornog na korozijumm/rev feed and cut depth of a p = 2 mm, the greatest tool life values were observed for v c= 50 m/min and v c = 150 m/min and they amounted to T = 44 min and T = 24 min. The minimum value of the tool life T = 19 min was v c = 118 m/min.Figure 3 Tool life for centre point parameters (T1)Figure 4 Tool life in dry machining of DSS with coated carbide tools T1Analysing the impact of cutting speed onto the tool life for a T1 tool point (Fig. 4) and T2 tool point (Fig. 5), it can be noticed that with the increasing cutting speed the tool life decreases for each of the feeds. The tool life decreases for the cutting speed of 100 to 130 m/min depending on the feed value. The higher the feed, the less the function moves to the v c axis increasing its value. Tool life takes larger values for the T1 tool point. The reason for this is probably a greater resistance to abrasive wear of tools with an Al2O3 coating.Figure 5 Tool life recorded in dry machining of DSS with coatedcarbide tools T23.3 Metallographic structureIn Figs. 6 to 11 metallographic structures of surface layer of DSS are presented and shown in 100× and 200× magnification in each case.All the figures show correct metallographic structure of duplex stainless steel i.e. ferrite and austenite. One can also see that no secretions that could arise between the grains of the two phases have appeared. The influence of temperature is not visible in the photos of the metallographic structure, probably for two reasons: the machining temperature has not exceeded 300 ÷ 450 °C or the exposure of the samples to the temperature above 300 °C has not been long enough to cause secretions between the grains of austenite and ferrite. The samples under investigation have been made of 1.4462 steel which contains from 0,08 to 0,20 % of nitrogen. Nitrogen added to duplex stainless steel causes higher stability of austenite and reduces the rate of secretion of disadvantageous intermetallic phases. Secondary phases have disadvantageous influence on mechanical properties and on corrosion resistance. The above mentioned properties of duplex stainless steel are the reason for its increased application. It should also be kept in mind, however, that duplex stainless steel has intermetallic phases (σ, χ) rich in Cr and Mo, which precipitate in ferrite. The sigma phase σ and the chi chase χ reduce the pinhole corrosion resistance and the intercrystallic corrosion resistance. They also cause the increase of brittleness. What is more, the authors of works [16] and [17] have found microhardness changes of the phases after machining in the process of turning super duplex stainless steel. This is related to the mechanisms of work hardening of the phases in the top layer. Deformation of austenite takes place as a result of grain contour rearrangement and has the character of plastic flow. SuchEffect of the cutting parameters impact on tool life in duplex stainless steel turning process G. Królczyk et al.rearrangement depends on the time of machining influence. In the light of the statements above, execution of further investigation aiming at the identification of the DSS top layer features, particularly microhardness, seems necessary.Figure 6 Surface layer metallographic structure of DSS after turning with tool T1: v c = 100 m/min, f = 0,3 mm/rev, a p = 2 mm, dry Figure 7 Surface layer metallographic structure of DSS after turning with tool T1: v c = 100 m/min, f = 0,3 mm/rev, a p = 2 mm, wet Figure 8 Surface layer metallographic structure of DSS after turning with tool T1: v c = 150 m/min, f = 0,3 mm/rev, a p = 2 mm, dry Figure 9 Surface layer metallographic structure of DSS after turning with tool T1: v c = 50 m/min, f = 0,3 mm/rev, a p = 2 mm, dryG. Królczyk et al. Utjecaj parametara obrade na vijek alata u procesu tokarenja dupleks čelika otpornog na korozijuFigure 10 Surface layer metallographic structure of DSS after turning with tool T2: v c = 100 m/min, f = 0,3 mm/rev, a p = 2 mm, dryFigure 11 Surface layer metallographic structure of DSS after turning with tool T2: v c= 100 m/min, f = 0,3 mm/rev, a p= 2 mm, wet4 ConclusionsDuring duplex stainless steel turning, the following difficulties occur: it is difficult to control the chip, there are excessive thermal and mechanical loads onto the tool point, strong adhesive interaction leading to the formation of built-up edge occur, and accelerated wear of cutting edge happens. These lead to:I.In the process of DSS turning the course of coatedcarbide tool point wear for the parameters of a test centre program shows a typical shape of the normal wear curve.II.Increasing the cutting speed increases the intensity of wear of the cutting edge.III.CVD - Ti(C,N)/Al2O3/TiN coated carbide tools indicate higher resistance to abrasive wear and they can be recommended to roughing machining of DSS, optimally with cutting speeds of 130 ÷ 150 m/min. IV.In the process of DSS turning no effect has been found of cutting speed and cooling on metallographic structure.5 References[1] Paro, J.; Hanninen, H.; Kauppinen, V. Tool wear andmachinability of X5 CrMnN 18 18 stainless steels. // Journal of Materials Processing Technology. 119, 1(2001), pp. 14-20.[2] Akasawa, T.; Sakurai, H.; Nakamura, M.; Tanaka, T.;Takano, K. Effects of free-cutting additives on the machinability of austenitic stainless steels. // Journal of Materials Processing Technology, vol. 143-144, (2003), pp.66-71.[3] Abou-El-Hossein, K. A.; Yahya, Z. High-speed end-millingof AISI 304 stainless steels using new geometrically developed carbide inserts. // Journal of Materials Processing Technology. vol. 162-163, (2005), pp. 596-602. [4] Charles, J. Austenitic Chromium – Manganese StainlessSteel – A European Approach. // Materials and Applications Series. vol. 12. Euro Inox, 2010.[5] Kosmač, A. Electropolishing Stainless Steel. // Materialsand Applications Series. vol. 11. Euro Inox, 2010.[6] Ciftci, I. Machining of Austenitic Stainless Steels usingCVD Multi-layer Coated Cemented Carbide Tools. //Tribology International. 39, (2006), pp. 565-569.[7] Cunat, P. J. The Euro Inox Handbook of Stainless Steel. //Materials and Applications Series. vol. 1. Euro Inox 2002. [8] Cunat, P. J. Working with Stainless Steel. // Materials andApplications Series. vol. 2. EDP Sciences and Euro Inox.2009.[9] Bouzid Saï, J.; Lebrun, L. Influence of Finishing byBurnishing on Surface Characteristics. // Journal of Materials Engineering and Performance. 12, 1(2003), pp.37-40.[10] Gunn, R. N. Duplex Stainless Steels: Microstructure,Properties and Applications. Abington Publishing,Cambridge, England 1997.[11] Grzesik, W. Advanced Machining Processes of MetallicMaterials. Theory, Modeling and Applications. ElsevierScience, 2008.[12] Polański, Z. Metody optymalizacji w technologii maszyn.PWN, Warszawa 1977.[13] Krolczyk, G.; Legutko, S.; Gajek, M. Predicting the surfaceroughness in the dry machining of duplex stainless steel. //Metalurgija. 52, 2(2013), pp. 259-262.[14] Krolczyk, G.; Gajek, M.; Legutko, S. Predicting the toollife in the dry machining of duplex stainless steel. // Eksploatacja i Niezawodnosc – Maintenance and Reliability. 15, 1(2013), pp. 62-65.[15] Montgomery, D. Design and Analysis of Experiments. 5thEdition. John Wiley & Sons Inc., New York, 2003.[16] Bordinassi, C. E.; Filho, O. C.; Delijaicov, S.; Batalha, F.G.; Stipkovic, F. M. Study of the Surface Integrity in aSuper Duplex Stainless Steel after Turning. 4º Congresso Brasileiro de Engenharia de Fabricação. Brazil, 2007. [17] Bordinassi, C. E.; Stipkovic, F. M.; Batalha, F. G.;Delijaicov, S.; de Lima, B. N. Superficial Integrity Analysisin a Superduplex Stainless Steel after Turning. // Journal ofEffect of the cutting parameters impact on tool life in duplex stainless steel turning process G. Królczyk et al.Achievements in Materials and Manufacturing Engineering.18, (2006), pp. 335-338.[18] Królczyk, G.; Legutko, S.; Raos, P. Cutting wedge wearexamination during turning of duplex stainless steel. //Tehnicki vjesnik-Technical Gazette. 20, 3(2013), pp. 413-418.Symbols and abbreviationsa p – depth of cut in mmf – feed rate in mm/revv c – cutting speed in m/minT – tool life in minVB B – width of flank wear in mmVB Bmax – the maximum width of the flank wear in mmDSS – Duplex Stainless SteelAuthors’ addressesGrzegorz Królczyk PhD. Eng.Faculty of Production Engineering and LogisticsOpole University of Technology76 Prószkowska Street, 45-758 Opole, PolandE-mail: g.krolczyk@po.opole.plMaksymilian Gajek Prof. DSc. PhD. Eng.Faculty of Production Engineering and LogisticsOpole University of Technology76 Prószkowska Street, 45-758 Opole, PolandE-mail: m.gajek@po.opole.plStanislaw Legutko Prof. DSc. PhD. Eng., Prof. h. c.Faculty of Mechanical Engineering and ManagementPoznan University of Technology3 Piotrowo Street, 60-965 Poznan, PolandE-mail: stanislaw.legutko@put.poznan.pl。
钻削精密深孔扭振发生装置的设计
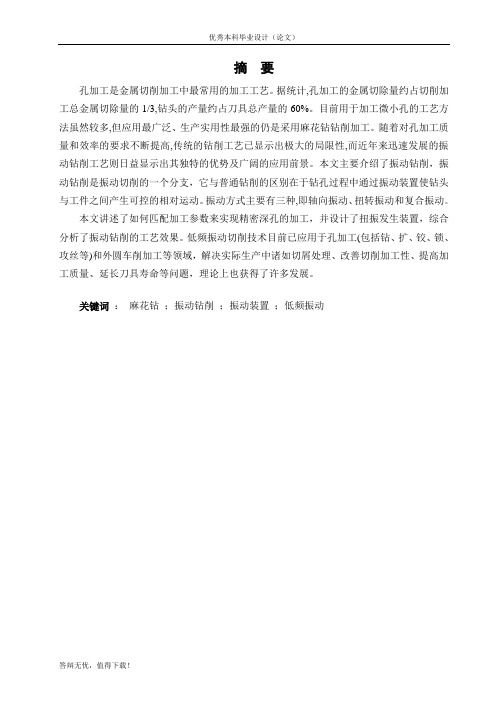
摘要孔加工是金属切削加工中最常用的加工工艺。
据统计,孔加工的金属切除量约占切削加工总金属切除量的1/3,钻头的产量约占刀具总产量的60%。
目前用于加工微小孔的工艺方法虽然较多,但应用最广泛、生产实用性最强的仍是采用麻花钻钻削加工。
随着对孔加工质量和效率的要求不断提高,传统的钻削工艺已显示出极大的局限性,而近年来迅速发展的振动钻削工艺则日益显示出其独特的优势及广阔的应用前景。
本文主要介绍了振动钻削,振动钻削是振动切削的一个分支,它与普通钻削的区别在于钻孔过程中通过振动装置使钻头与工件之间产生可控的相对运动。
振动方式主要有三种,即轴向振动、扭转振动和复合振动。
本文讲述了如何匹配加工参数来实现精密深孔的加工,并设计了扭振发生装置,综合分析了振动钻削的工艺效果。
低频振动切削技术目前已应用于孔加工(包括钻、扩、铰、锁、攻丝等)和外圆车削加工等领域,解决实际生产中诸如切屑处理、改善切削加工性、提高加工质量、延长刀具寿命等问题,理论上也获得了许多发展。
关键词:麻花钻;振动钻削;振动装置;低频振动AbstractHole processing is the most commonly used metal cutting machining processing technology. According to statistics, hole machining of metal removal accounted for about one-third of the total machining metal removal of the, drill production accounted for about 60% of the total tool production. Process methods now used for machining small holes while more, but the strongest is still the most widely used, the production practicality is uses the twist drill drilling processing. As the hole of the requirement of increasing the quality and efficiency, the traditional drilling technology has shown great limitations, in recent years the rapid development of the vibration drilling technology is increasingly shows its unique advantages and broad application prospects.Vibration drilling is mainly introduced in this paper, the vibration drilling is a branch of vibration cutting, the difference between it and common drilling through vibration device in the process of drilling bit and generate controllable relative movement between parts. Vibration mode mainly has three kinds, namely axial vibration, torsional vibration, and vibration compound.This article tells the story of how the matching processing parameters to achieve precision deep hole machining, and torsional vibration generator is designed, the comprehensive analysis of the vibration drilling technology effect. Low frequency vibration cutting technology has been applied to the machining (including drilling, expanding, hinge, lock, tapping, etc.) and cylindrical turning processing, etc, to solve practical production in cutting machining, such as chip removal, improve processing quality, prolong tool life and other issues, theory also received many development.Keywords:Twist drill ;Vibration drilling;Vibration device;Low frequency vibration目录摘要 (I)Abstract (II)目录........................................................................................................................................... V I 1 绪论 (1)1.1 振动钻削技术的发展历史 (1)1.2 振动钻削的工艺效果 (2)1.3 振动钻削的应用前景及前沿课题 (4)2 振动钻削的原理 (7)2.1 振动钻削的机理 (7)2.2振动钻削系统的稳定性与振幅损失 (8)2.2.1 振动钻削时的切削力 (9)2.2.2 振动钻削系统的稳定性 (10)2.2.3 产生横向摆振与钻杆弯曲振动的原因 (12)2.2.4 振幅损失 (13)3 深孔加工的高效解决方案 (14)3.1深孔加工 (14)4装置设计 (16)4.1装置总体方案 (16)4.2电机的选择 (18)4.3带传动设计 (20)4.3.1 确定计算功率Pca (20)4.3.2选择带型 (20)4.3.3 确定带轮的基准直径 (20)4.3.4 确定中心距a和带的基准长度Ld (21)4.3.5验算主动轮上的包角α1 (21)4.3.6确定带的根数Z (21)4.3.7确定带的预紧力F0 (22)4.3.8计算带传动作用在轴上的力(简称压轴力)F p (22)4.3.9 V带轮设计 (22)4.3.10 V带传动的张紧装置 (23)4.4偏心轴及其附件设计 (24)4.4.1 轴承的选用 (26)4.4.2轴承底座 (27)4.4.3 端盖和透盖 (28)4.4.4 偏心销钉 (29)4.5主轴及其附件设计 (29)4.5.1 主轴 (29)4.5.2 弹性夹头 (30)4.5.3 轴承的选用 (31)4.5.4 轴承座 (32)4.5.5 夹紧螺母 (32)4.5.6 轴承盖 (33)4.5.7 摆杆 (33)4.6底板设计 (33)5 致谢 (35)参考文献 (36)1 绪论1.1 振动钻削技术的发展历史据统计,孔加工是金属切削加工中最重要的工序之一。
大面积微结构辊对板热压印装置研发及工艺优化
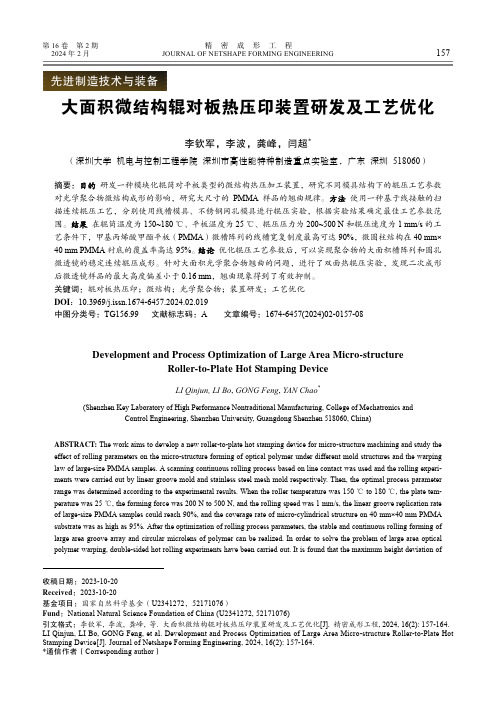
第16卷 第2期 精 密 成 形 工 程收稿日期:2023-10-20 Received :2023-10-20基金项目:国家自然科学基金(U2341272,52171076)Fund :National Natural Science Foundation of China (U2341272, 52171076)引文格式:李钦军, 李波, 龚峰, 等. 大面积微结构辊对板热压印装置研发及工艺优化[J]. 精密成形工程, 2024, 16(2): 157-164. LI Qinjun, LI Bo, GONG Feng, et al. Development and Process Optimization of Large Area Micro-structure Roller-to-Plate Hot Stamping Device[J]. Journal of Netshape Forming Engineering, 2024, 16(2): 157-164. *通信作者(Corresponding author )李钦军,李波,龚峰,闫超*(深圳大学 机电与控制工程学院 深圳市高性能特种制造重点实验室,广东 深圳 518060) 摘要:目的 研发一种模块化辊筒对平板类型的微结构热压加工装置,研究不同模具结构下的辊压工艺参数对光学聚合物微结构成形的影响,研究大尺寸的PMMA 样品的翘曲规律。
方法 使用一种基于线接触的扫描连续辊压工艺,分别使用线槽模具、不锈钢网孔模具进行辊压实验,根据实验结果确定最佳工艺参数范围。
结果 在辊筒温度为150~180 ℃、平板温度为25 ℃、辊压压力为200~500 N 和辊压速度为1 mm/s 的工艺条件下,甲基丙烯酸甲酯平板(PMMA )微槽阵列的线槽宽复制度最高可达90%,微圆柱结构在40 mm× 40 mm PMMA 衬底的覆盖率高达95%。
车工实习加工工艺流程
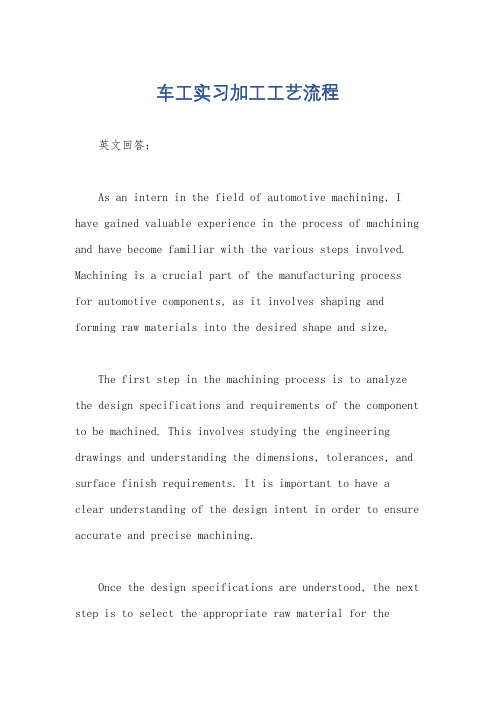
车工实习加工工艺流程英文回答:As an intern in the field of automotive machining, I have gained valuable experience in the process of machining and have become familiar with the various steps involved. Machining is a crucial part of the manufacturing processfor automotive components, as it involves shaping and forming raw materials into the desired shape and size.The first step in the machining process is to analyze the design specifications and requirements of the component to be machined. This involves studying the engineering drawings and understanding the dimensions, tolerances, and surface finish requirements. It is important to have aclear understanding of the design intent in order to ensure accurate and precise machining.Once the design specifications are understood, the next step is to select the appropriate raw material for thecomponent. This could be a metal alloy, such as aluminum or steel, or a composite material. The choice of material depends on factors such as strength, durability, and cost.It is important to consider the properties of the material and how it will behave during the machining process.After selecting the raw material, the next step is toset up the machining equipment. This involves preparing the machine tools, such as lathes, milling machines, or CNC machines, and ensuring that they are properly calibratedand in good working condition. The cutting tools, such as drills, end mills, or inserts, also need to be selected and installed correctly.Once the equipment is set up, the actual machining process begins. This typically involves a series of operations, such as turning, milling, drilling, or grinding, depending on the design requirements. Each operation is performed with precision and accuracy to ensure that the component is machined to the desired dimensions and surface finish.During the machining process, it is important to monitor the cutting conditions, such as cutting speed, feed rate, and depth of cut. This helps to optimize the machining parameters and ensure efficient material removal. It is also important to use proper cutting fluids or lubricants to reduce friction and heat generation, which can affect the quality of the machined surface.After the machining process is complete, the next step is to inspect the machined component for any defects or deviations from the design specifications. This could involve using measuring instruments, such as calipers, micrometers, or coordinate measuring machines, to check the dimensions and tolerances. Any necessary adjustments or corrections can then be made to ensure that the component meets the required standards.In conclusion, the process of machining in automotive manufacturing involves analyzing design specifications, selecting raw materials, setting up the equipment, performing machining operations, monitoring cutting conditions, and inspecting the final product. This processrequires a combination of technical skills, attention to detail, and precision to produce high-quality automotive components.中文回答:作为一名汽车加工实习生,我在加工过程中积累了宝贵的经验,并熟悉了其中的各个步骤。
机加工作业指导书标准范文
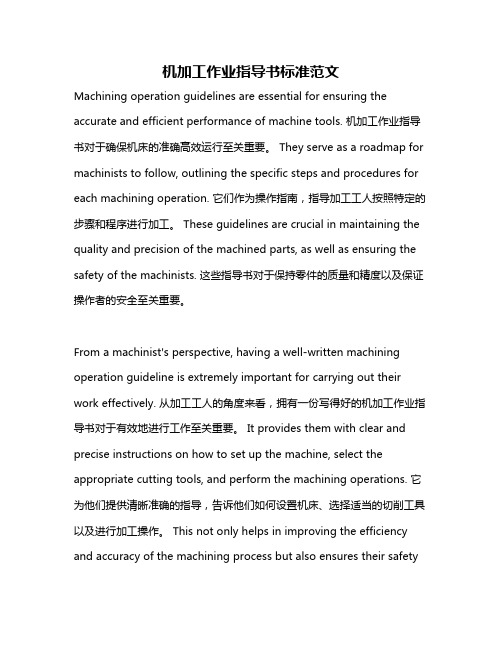
机加工作业指导书标准范文Machining operation guidelines are essential for ensuring the accurate and efficient performance of machine tools. 机加工作业指导书对于确保机床的准确高效运行至关重要。
They serve as a roadmap for machinists to follow, outlining the specific steps and procedures for each machining operation. 它们作为操作指南,指导加工工人按照特定的步骤和程序进行加工。
These guidelines are crucial in maintaining the quality and precision of the machined parts, as well as ensuring the safety of the machinists. 这些指导书对于保持零件的质量和精度以及保证操作者的安全至关重要。
From a machinist's perspective, having a well-written machining operation guideline is extremely important for carrying out their work effectively. 从加工工人的角度来看,拥有一份写得好的机加工作业指导书对于有效地进行工作至关重要。
It provides them with clear and precise instructions on how to set up the machine, select the appropriate cutting tools, and perform the machining operations. 它为他们提供清晰准确的指导,告诉他们如何设置机床、选择适当的切削工具以及进行加工操作。
机械加工工艺优化创新案例范文

机械加工工艺优化创新案例范文## English Answer:Innovative Case Study of Mechanical Processing Technology Optimization.Introduction.Mechanical processing is a key process in the manufacturing industry, and its efficiency and quality directly affect the overall production efficiency and product quality. With the continuous advancement of manufacturing technology, the optimization and innovation of mechanical processing technology have become important directions for improving manufacturing capabilities.Case Study.In a manufacturing enterprise, the traditional mechanical processing technology of a certain component hadthe following problems: low processing efficiency, high defect rate, and unstable product quality. To solve these problems, the enterprise conducted a comprehensive optimization and innovation of the mechanical processing technology.Optimization Measures.1. Tool Optimization: Optimized the selection ofcutting tools, using high-quality coated tools with appropriate geometry and materials to improve cutting efficiency and reduce tool wear.2. Process Parameter Optimization: Adjusted the cutting parameters, such as cutting speed, feed rate, and depth of cut, based on the material properties and tool characteristics to achieve optimal cutting performance.3. Machining Strategy Optimization: Improved the machining strategy, such as using trochoidal milling and high-speed machining, to reduce machining time and improve surface quality.4. Quality Control Optimization: Implemented strict quality control measures, including real-time monitoring of machining parameters and regular inspection of product quality, to ensure the stability and accuracy of the machining process.Innovation Measures.1. Application of Intelligent Manufacturing Technology: Introduced intelligent manufacturing technology, such as machine vision and adaptive control, to automate the machining process, improve processing accuracy, and reduce operator dependence.2. Development of New Machining Methods: Explored and developed new machining methods, such as ultrasonic machining and laser machining, to meet the processing requirements of special materials and complex geometries.3. Optimization of Fixture Design: Optimized the design of fixtures and clamping methods to improve workpiecestability, reduce machining deformation, and ensure processing accuracy.Results.After implementing the optimization and innovation measures, the mechanical processing technology of the component was significantly improved:1. Processing efficiency increased by 25%.2. Defect rate decreased by 40%.3. Product quality stability improved significantly.4. Manufacturing costs were reduced by 15%.Conclusion.Through the optimization and innovation of mechanical processing technology, the enterprise achieved significant improvements in processing efficiency, defect rate, productquality, and manufacturing costs. The case study demonstrated the importance of continuously improving mechanical processing technology to enhance manufacturing capabilities and achieve sustainable development.## 中文回答:机械加工工艺优化创新案例范文。
Effects of processing parameters on the mechanical properties

Material PropertiesEffects of processing parameters on the mechanical propertiesof polypropylene random copolymerSenol Sahin,Pasa Yayla*Mechanical Engineering Department,Engineering Faculty,Kocaeli University,41040Kocaeli,TurkeyReceived9June2005;accepted19July2005AbstractThe mechanical properties of polypropylene random copolymer(PP-R)with different processing parameters were studied. Special attention is devoted to the investigation of the influence of masterbatch addition on the variation in the mechanical properties of injection moulded PP-R.Tensile,instrumented Charpy impact,Shore D hardness,differential scanning calorimeter(DSC)and Vicat softening temperature(VST)tests were conducted on the test samples containing different colour masterbatches varying from0.5to10wt%.The observed changes in the mechanical behaviour are explained by the type and level of masterbatch content.The natural UV weathering performance of the PP-R material was studied from the masterbatch type point of view.The effect of processing parameters on material performance was studied on samples which were directly obtained from extruded pipes and on injection moulded samples.Finally,the effects of storage time on the polymer properties were investigated.q2005Elsevier Ltd.All rights reserved.Keywords:Polypropylene random copolymer;Processing parameters;Masterbatch types;Masterbatch contents;Ultraviolet degradation; Storage time1.IntroductionThe use of plastic materials in pipe applications is well established because of the lightweight,high performance, and excellent corrosion performance they can offer compared with metallic materials such as iron and copper. Having achieved high level of penetration in different applications,varying from water supply to gas distribution networks,from sanitary and heating systems to waste water collection and discharge systems,the use of plastics is expected to continue growing steadily at a rate of about5–10%per year[1].In many applications it is necessary to pigment the resin to specific colour for modifying the optical appearance for design,styling and functional purposes.Because of the importance of pigment addition to the base polymer,many studies[2,3]have been undertaken to understand their effects on the performance of plastics,especially since it has been shown that incorporating additives into polymeric materials during fabrication often affects rheological[4], mechanical[5,6]and optical properties[7]in an unpredict-able,and sometimes detrimental manner.PP can be coloured by two different methods.In thefirst method, certain types of colouring pigments are added to the natural base polymer at a certain pre-defined percent by the converters during either injection moulding or extrusion of thefinal product.In the second method,the base polymer is coloured by the raw material producer by compounding during the production process.Since the second method gives more uniform colouring,both the raw material producers and,generally,the end-users prefer compounded polymer.The polymer producers claim thatthe *Corresponding author.Tel.:C902623351148;fax:C902623352812.E-mail address:pyayla@.tr(P.Yayla).development of coloured PP-R raw material has to be done by the polymer producers due to the fact that specifically designed compounding equipment is used to obtain proper pigment and additive distribution without damaging the molecular structure[8].Furthermore,it is generally claimed that coloration with masterbatches done by the converters leads in general to poor pigment dispersion,resulting in pigment agglomerates acting as defects in the polymer matrix and impairing the mechanical properties of the finished product.In fact,when a colour masterbatch is used during the converting,the distribution level achieved in the extruder is usually not acceptable,resulting in uneven distribution[8].Despite these disadvantages,colouring by the polymer converter is cheaper and gives them some additional logistic advantages.Moreover,it is fairly seldom that a specific colorant is added to the reactants during a polymerisation process,unless such polymer resin is required in a large volume.Polypropylene random copolymer(PP-R)is one of the fastest growing of plastics being used in sanitary and heating applications.The overall mechanical properties of PP are strongly influenced by testing and processing parameters of the polymer.Hence,knowledge of relations between structure and mechanical properties of polymers enables the manufacturers to produce materials with certain morphologies by altering the processing conditions[9]. The influence of testing parameters on the overall performance was discussed in Part1of this work[10]. Determining the effects of processing parameters on the mechanical properties of PP-R is the objective of this study. Special attention is devoted to the investigation of influence of masterbatch addition on the variation in the mechanical properties.2.Experiments2.1.MaterialsThe base polymer used in this study is a natural colour polypropylene random copolymer(PP-R),produced by Borealis S.A.,trade name Borealis RA130 E.The properties of this natural PP-R are given in the work by Sahin and Yayla[10].It is known that colouring in general and the method of colouring in particular,might have some influence on the mechanical properties of the polymer[11].In this study,the effect of colouring methods on the overall mechanical performance of PP-R is investigated.In addition to the method of colouring,the types of pigment used for colouring could influence the overall mechanical performance of the material.In general,inorganic pigments yield more stable and better mechanical properties than organic anic pigments, however,are known for their high colour strength, brightness and good transparency[12].In order to determine the effects of different colouring on material properties,four different colour masterbatches were used.The masterbatches used to make thefinal product in different colours were supplied by local masterbatch manufacturers.They were supplied in granule form and the carrier resin for pigments for the all types of masterbatches was PP-R.The typical properties of masterbatches used in this study are detailed in Table1.Four different coloured PP-R materials,compounded during polymerisation by the above named producer,were used to prepare test samples.From the producers’point of view,there is no significant difference in the typical properties of these different colour compounds.2.2.Specimen preparationFour different groups of samples were prepared in this investigation.For thefirst group,an un-compounded natural material was coloured during the injection process. For this group of samples,different types of masterbatches at pre-defined ratios,varied between0.5,1,2.5,5and 10%in weight,were added during the injection process of the test samples.Before introducing the resin and masterbatches into the injection moulding machine,the masterbatch and the resin were put in a mixer and mixed for about30min.For the second group,a compounded PP-R material in blue,white,grey and green was used to prepare test samples by injection moulding.For these two groups,the detailed information on the injection mould and injection parameters was given elsewhere[10].For the third and fourth groups,the test samples were directly cut and extracted from the extruded pipe manufactured from white colour compounded,and1%white colour masterbatch added materials,respectively.These third andTable1Typical properties of masterbatches used in this studyM1M2M3M4 Colour White Green Blue Black Pigment type Organic Organic/Inorganic Organic/Inorganic Inorganic Total pigment concentration(%)60503040Meltflow index(gr/10min) (2308C,21.2N)6.017.934.1!0.01S.Sahin,P.Yayla/Polymer Testing24(2005)1012–10211013fourth groups of samples are coded as W1and W2,respectively.3.Mechanical testsUnless otherwise mentioned,all tensile tests were carried out at a crosshead speed of 50mm/min and,before testing,all samples were conditioned at room temperature for a period of 30days.All the results are average of three tests.The effects of processing parameters,masterbatch types and content and natural UV weathering on the properties of material were monitored using tensile,Charpy impact,Shore D hardness,and DSC tests.The details on these three tests were outlined elsewhere [10].3.1.Microstructural analysisAs the addition of any types of additives may alter the crystallisation characteristics of PP [13],a differential scanning calorimeter (DSC)analysis was used to evaluate thermal and morphological characteristics and the degree of crystallinity in the moulded samples containing different types of masterbatches at different concentrations.Tests were carried out on a Rheometric Scientific Polymer Laboratories instrument.Samples,each having a weight of about 13mg,were extracted from the middle sections of injection moulded samples,shown in Fig.1(c)of Sahin and Yayla [10].In the DSC tests,each sample was heated from 30to 2008C at a rate of 108C/min under a nitrogen atmosphere.Both thermal and crystallisation parameters were obtained from the heating scans.The level of crystallinity was calculated with the Eq.(1).c ZD H scc100(1)where D H sc is the melting enthalpy of the semi-crystalline material to be studied,and D H c is the enthalpy of 100%crystalline material.Since,a specific value of D H c for PP-Rdoes not exist,and the enthalpy of fusion for 100%crystalline polypropylene is almost independent of the isotacticity and equals 207J/g [14],the value of D H c for PP-R is taken as 207J/g.The melting temperature was taken as the peak temperature in the curves.The peak area,calculated automatically by the DSC instrument,was taken as the melting enthalpy.Typical thermal histories of some PP-R samples are given in Fig.1.It is evident that there is an endothermic melting peak in the heating scans.From the thermograms,it could be deduced that the onset of melting temperature is around 1108C and the melting point,the maximum of endothermic of melting peak in the scan,is around 1458C.From the figure,the crystallinity could be calculated as 30%.It has been demonstrated that there is very little difference between samples containing the natural and 1%of different colour masterbatches.Another important feature to be considered in Fig.1is that the DSC scans of all samples,namely natural,1%white colour masterbatch containing PP-R,white compound,W1and W2samples differ remarkably from each other,not only in their overall history but also in their melting temperature peaks.These variations are mainly due to the method that the samples are coloured,pounding or 1%masterbatch addition,and the way the samples are prepared,i.e.injection or extrusion.The cooling history of the extruded pipes and injected samples is rather different,resulting in some morphological variations in the samples.The early peaks and other multiple peak phenomena in the DSC tests are attributed to the compositional hetero-geneity of the crystal morphology of the polymer [15,16],and to lower-molecular weight polymer,which melted very early (i.e.wax,processing aid,dispersion aid)[17].3.2.Colouring effectsThe colouring of plastic products can be achieved easily by adding a small percentage of colour masterbatches during processing.To determine the effects of masterbatch concentration on the tensile properties of PP-R,tensile tests on samples containing 0.5,1,2.5,5and 10%masterbatches,detailed in Table 1,were carried out.Fig.2depicts the variation of yield stress with different colour masterbatch content.The figure shows that the addition of masterbatch diminishes the yield stress for masterbatch contents of up to about 0.5%.Moreover,the yield stress recovers with increasing the masterbatch content,and increasing the content enhances the yield stress as well.It is worth pointing out that the yield stresses of white,green and blue colour compounds are very comparable and more or less similar to that of 0.5%masterbatch samples.The decrease in the yield stress with masterbatch content is due to the high MFI value of the masterbatch (see Table 1).On the other hand,the recovery and increase in the yield stress is attributed to the reinforcing effect of pigments in the masterbatch [11],as well as the nucleating effects of these pigments [18,19].203550658095110125140155170185–1.8–1.5–1.2–0.9–0.6–0.30.00.3 Natural 1% White White W2 W1H e a t F l o w [m c a l /s ]Temperature [°C]Fig.1.DSC thermal history of natural and coloured PP-R showing remarkable influence of the method of colouring and the way the sample is prepared.S.Sahin,P.Yayla /Polymer Testing 24(2005)1012–10211014The last point in Fig.2to be mentioned is that the yield stresses of samples extracted from the pipe,namely W1and W2,are significantly lower than that of the injection moulded test samples.The difference between W1and W2is thought to be mainly due to the method of colouring,and the differences between W1,W2and others are due to differences between the crystallisation histories of injection and extrusion of the samples.This is another illustrative example of the strong interrelationship between structure,processing,morphology and final polymer [20].Fig.3shows the variation of yield strain with masterbatch content of the samples.The figure clearly reveals that adding the masterbatch reduces the yield strain,and this decline is almost linear for all masterbatch types.The yield strain also depends on colour compound and is much lower for the samples extracted directly from the pipe.The decline in yield strain as a function of masterbatch content is attributed to the reinforcing effect of pigments in the masterbatches.Y i e l d S t r e s s [N /m m 2]Masterbatch Content [%]Fig.2.Variation of yield stress with masterbatch contents for PP-R material.(The bars W,white colour compound;GN,green colour compound;B,blue colour compound;GR,grey colour compound;W1,White colour compound pipe;W2,1%white colour masterbatch containing pipe).Y i e l d S t r a i n [%]Masterbatch Content [%]Fig.3.Variation of yield strain with masterbatch contents for PP-R material.S.Sahin,P.Yayla /Polymer Testing 24(2005)1012–10211015The variation of Charpy impact energy with masterbatch content is shown in Fig.4,showing that for all types of masterbatches the total Charpy impact energies increase with masterbatch content up to 1%,after which the impact energy remains constant.However,the impact energy increases almost linearly with the white masterbatch content.Regarding the compounded samples,the green and blue compounded samples showed the highest impact resistance.Fig.4indicates more clearly that the inorganic based pigments (namely green,blue,and black compounds and masterbatch added samples)give better impactproperties than organic compounds (namely white,PP-R polymer).As pointed out elsewhere [10],the Charpy impact crack initiation and propagation resistance of the material are rather sensitive to the test temperature.The lower transition temperature is around 08C,and above 858C the material becomes too ductile to break.The present investigation made it clear that neither the content nor the type of masterbatch had any effect on this brittle-ductile transition.The effect of masterbatch content on melting tempera-ture,extracted from the DSC scans,is shown in Fig.5indicating that,except for the white masterbatch,addingC V [k J /m 2]Masterbatch Content [%]Fig.4.Variationof Charpy impact energy (C v )with masterbatch contents for PP-R material.M e l t i n g P o i n t [°C ]Masterbatch Content [%]Fig.5.Variation of melting point [T m ]with masterbatch contents for PP-R material.S.Sahin,P.Yayla /Polymer Testing 24(2005)1012–10211016masterbatch does not have a significant influence on the melting point of the polymer.The melting points of coloured and compounded samples do not differ signifi-cantly from each other.Fig.6indicates the volume percentage of crystallinity of compounded and coloured PP-R samples.It could be concluded from the figure that the addition of masterbatch and compounding diminishes the crystallinity and the level of crystallinity differs from one masterbatch to another,which could be attributed to the different level of nucleating activity of each pigment [18].Therefore,different types of pigments incorporated in PP-R result in different degrees of crystallisation.These results agree with the findings of Kening et al.[21]and Krisher and Marshall [22],which showed that incorporating pigments into PP affected its mechanical properties mainly in a positive fashion.3.3.Time effectsIt is known that the properties of the polymeric material may significantly change just after conversion,i.e.by extrusion or injection processes.However,the history of this property change is not known for this material,so it is worth investigating what changes take place and how they depend on time.After injection moulding,the PP-R samples were conditioned at 228C and 50%relative humidity for a wide range of times—between 5min and 23months—in a box which excluded light.Fig.7shows the effect of storage time on yield strength and Charpy impact resistance of 1%white and 1%green masterbatch containing PP-R material.It is seen that the impact strength decreases considerably with storage time,and that it stabilizes about 30days after production.As for the yield stress,Fig.7shows that the yield stress increases gradually with storage time and that after around 30days itc [%]Masterbatch Content [ % ]Fig.6.Variation of crystallinity with masterbatch contents for PP-R material.05101520253035Conditioning Time [hour]σy [N /m m 2]C V [k J /m 2]Fig.7.Effects of storage time on yield stress (s y )and Charpy impact energy (C v )of 1%white and 1%green masterbatch containing PP-R material.Conditioning Time [hour]T m [°C ]c [%]Fig.8.Effect of storage time on melting temperature (T m )and crystallinity (c)of natural PP-R material.S.Sahin,P.Yayla /Polymer Testing 24(2005)1012–10211017remains fairly unchanged.The figure also reveals that time dependent tensile strength and impact resistance values are not sensitive to the type of masterbatch.The variation of melting temperature and crystallinity with storage time is shown given in Fig.8,indicating that both crystallinity and melting temperature increase with storage time and that both values stabilise after about 30days.Similarly,the variation of Shore D hardness with storage time is plotted in Fig.9,pointing out that the hardness increases gradually after the injection of the samples and remains relatively unchanged after more than 30days after production.Vicat softening temperature tests (VST)were utilised to determine the softening temperature of the material.A Zwick Vicat softening temperature tester at 50N force and 508C/h heating rate was utilised to determine temperature at which the indentor penetrates 1mm into the material.This value is particularly important for the conversion of the material into the product.The variation of VST with storage time is given in Fig.10,showing a minor increase with conditioning time for natural PP-R.3.4.Natural UV weatheringEvery polymeric material exposed to direct sunlight undergoes some damage.Plastic pipes made from PP-R could be used outdoors and thus may undergo ultraviolet (UV)degradation.This is especially the case for PP-R pipes used in solar heating systems.For this type of application,it is particularly important to decide what colour of PP-R pipe to use and how its mechanical properties deteriorate with the UV exposure time.Exposure of many plastics to ultraviolet radiation causes a loss in their mechanical properties.The mechanical property most severely affected is usually theV S T [°C ]Conditioning Time [hour]Fig.10.Effect of storage time on VST for natural PP-R material.H a r d n e s s (S h o r e D )Conditioning Time [hour]Fig.9.Effect of storage time on Shore D hardness for natural and 1%w white masterbatch containing PP-R material.50010001500200025003000350040004500500055000.00.51.01.52.02.53.03.54.0Januar 02December T m 5.3°C ϕm 85.9%October T m 16.7°C ϕm 70.4%September T m 21.7°C ϕm 66.8%November T m 11.2°C ϕm 74.4%August T m 24.6°C ϕm 73.3%July T m26.1°Cϕm 68.6%June T m 22.5°C ϕm 60.3%A b s o r b e d T o t a l S o l a r R a d i a t i o n E n e r g y [G J /m 2]Solar Exposure Time [hour]Fig.11.Absorbed total solar radiation energy variation as a function of UV exposure time considered in this study.T m and 4m represent the average temperature and humidity,respectively.S.Sahin,P.Yayla /Polymer Testing 24(2005)1012–10211018ductility of the material [23].Pigment systems,on the other hand,influence to what extent materials are affected by ultraviolet radiation exposure.Some colours,such as black,may make the material less susceptible to ultraviolet radiation degradation than others [24].Several series of tensile,Charpy impact,Shore D and DSC tests were carried out on test samples exposed to sunshine for different times,up to six months during the summer time.All samples were suspended so that they could freely rotate,enabling each surface to be UV affected uniformly.Fig.11shows the total absorbed energy as a function of exposure time.The variation of tensile properties with exposure time is shown in Fig.12.The figure reveals that both yield stress and yield strain decreases with UV exposure time for all samples.Most significantly,the greatest decrease is seen in the natural PP-R.The least UV affected sample is the PP-R containing 1%black masterbatch.The effect of natural UV weathering time on the Charpy impact resistance of PP-R material is shown in Fig.13,showing that for all the samples the Charpy impact energydiminishes with the UV exposure time.However,black samples are the least and natural samples the most affected.Fig.14shows the variation of the Shore hardness with UV exposure time.The figure shows that just after the beginning of natural UV exposure,the hardness of the samples increases with exposure time for all samples.It is also worth mentioning that the least affected are the natural colour samples,which have higher opacities.The hardness increase in blue and green samples is pronounced.Black samples are exceptions,mainly due to the carbon black content of the masterbatch.As stated by Turton and White [24],pigments that simply reflect or scatter UV,preventing it from penetrating far into the plastics,also limit degradation to a region close to the surface.Fundamental studies in the area of polymer morphology almost universally employ DSC.Thermal analysis has also played a significant role in the degradation studies of semicrystalline polymers [25].DSC tests on UV degraded samples could be a good indication of morphologicalWeathering Time [hour]εy [%]σy [N /m m 2]Fig.12.Natural UV weathering time effect on yield stress (s y )and yield strain (3y )of natural and 1%coloured PP-R material.Weathering Time [hour]C V [k J /m 2]Fig.13.Natural UV weathering time effect on Charpy impact strength (C v )of natural 1%coloured PP-R material.Weathering Time [Hour]H a r d n e s s [S h o r e D ]Fig.14.Natural UV weathering time effect on Shore D hardness of natural and 1%coloured PP-R material.7085100115130145160175190–1.6–1.1–0.6–0.10.40.90 hour 504 hour 2304 hour 4776 hourH e a t F l o w [m c a l /s ]Temperature [°C]Fig.15.Natural UV weathering time effect on the DSC thermal history of natural PP-R material.S.Sahin,P.Yayla /Polymer Testing 24(2005)1012–10211019changes in the material.The DSC scans obtained in this study show that the degree of UV degradation sensitivity differs from one colour to other.From the comparison of Figs.15–17,it could be deduced that the most affected sample is the natural (Fig.15)and the least effected ones are the black,blue (Fig.16),white (Fig.17)and green coloured samples,respectively,showing strong structural modifi-cations due to UV degradation.This dependence is attributed to the fact that the different pigments used in the masterbatches have different UV absorption/screening mechanisms,resulting in different protective mechanisms against UV exposure [26,27].The effect of adding different colour masterbatches on the UV performance of PP-R material was also evaluated using the melt flow index (MFI)study.MFI was measured at 2308C at 21.6N according to ISO 1133standard on UV exposed samples containing 1wt%blue,black,green and white colour masterbatch.For the sake of comparison,the variation of MFI with exposure time for natural PP-R was also measured.The results in Fig.18show that natural andgreen samples were the most affected and black,blue and white samples were the least UV affected.The results of these MFI measurements correlate with the DSC results in Figs.15–17.The increase in MFI is attributed to the chain scission and formation of new groups [25].4.ConclusionsThe results of the present investigation indicate that the way the test samples are produced,i.e.injection or extrusion,strongly influences the overall mechanical properties of polymers.Mechanical and DSC tests showed that both the type and content of masterbatches in PP-R influence not only the degree of crystallinity but also the structure and mechanical properties of the polymer.The addition of colour masterbatches increases the short term yield stress and impact strength but reduces the yield strain .The yield and Charpy impact resistance of both natural and 1%masterbatch containing PP-R material continuously vary during the room temperature conditioning period of about 30days,and then remain fairly constant.The property changes in mechanical and thermal properties,particularly in yield strength and Charpy impact resistance,after the injection moulding could not solely be explained through ‘post-crystallisation’.Apart from the slight variation in crystallinity,the influence of the polymer parameters fixed in the synthesis,namely average molar mass,molar mass distribution and chain regularity [28]as well as the reduction of free volume of the amorphous phase has to be taken into account [29].For all naturally aged PP-R samples coloured in different ways the yield strength,yield strain and Charpy impact energy decrease with increasing natural UV weathering time.Shore D hardness,on the other hand,increased with the degradation time.Tensile and impact properties were affected mostly by UV in samples having higher opacities.7085100115130145160175190–1.7–1.5–1.3–1.1–0.9–0.7–0.5–0.30 hour 504 hour 2304 hour 4776 hour H e a t F l o w [m c a l /s ]Temperature [°C]Fig.16.Natural UV weathering time effect on the DSC thermal history of 1%blue coloured PP-R material.Weathering Time (hour)M F I (g r /10 m i n )Fig.18.Effect of natural UV weathering time on the variation of MFI values for PP-R material containing 1%white,green,blue and black masterbatch and natural PP-R.7090110130150170190–2.0–1.5–1.0–0.50.0504 hour 2880 hour 4320 hour H e a t F l o w [m c a l /s ]Temperature [°C]Fig.17.Effect of Natural UV weathering time on the variation of DSC melting curves for PP-R material containing 1%white masterbatch.S.Sahin,P.Yayla /Polymer Testing 24(2005)1012–10211020Morphology,crystallization and melting behaviour is also affected by the addition of masterbatch to a degree which depends on masterbatch type.This study provides a clear view of the influence of masterbatch type and content on both mechanical proper-ties.Similarly,the UV degradation of the PP-R is very much masterbatch,thus pigment,dependent,making it clear that pigments vary in their UV stability.With this work it is evidenced that the MFI tests on UV degraded samples are quite useful to assess the extent of degradation.This has practical implications for durability assessment and stabil-ization strategies.AcknowledgementsWe are greatly indebted to colleagues at both industrial as well as university laboratories for their positive interest, and especially to Turkkablo A.O.,Emas A.S.,and Pipelife A.S.The raw materials supplied by Borealis S.A.are gratefully acknowledged.Dr P.S.Leevers of Imperial College UK,and Prof.Dr E.Cavusoglu of Arili Plastik of Turkey are also appreciated for their valuable discussion and comments on the manuscript.References[1]G.Beer,Polypropylen(PP),Kunststoffe86(10)(1996)1460–1463.[2]R.L.Gray,R.E.Lee,Influence of co-additive interactions onstabilizer performance,ANTEC’96(1996)2683–2687. [3]J.N.Birmingham,Volatility of titanium dioxide pigments inpolyethylenefilm extrusions,ANTEC’95(1995)3290–3294.[4]E.E.Jaffe,C.D.Campell,S.B.Hendi,F.Babler,Rheologicallyeffective organic pigments,J.Coating Technol.66(1994)47–54.[5]M.C.Yu,M.A.Bissel,R.S.Whitehouse,Effect of carbonblack dispersion on polymer performance,ANTEC’95(1995) 3246–3250.[6]D.Williams,M.Bevis,The Effects of recycled plastics andcompound additives on the properties of injection-moulded polypropylene co-polymer,J.Mater.Sci.15(1980)2834–2842.[7]J.Spano,W.Steen,Pigment evaluation of talc in poly-propylene,ANTEC’97(1997)2711–2715.[8]R.Bresser,A.Sallaberger,PP-R for Demanding Plumbing andHeating Applications:Success Through High Quality Systems,Borealis Technical Publication,2001.[9]J.U.Strake,G.H.Michler,W.Grellman,S.Seider,M.Gahleithener,J.Fiebig,E.Nazbedova,Fracture toughness of polypropylene copolymers:influence of interparticle distance and temperature,Polymer39(1)(1998)75–82. [10]S.Sahin,P.Yayla,Effects of testing parameters on themechanical properties of polypropylene random copolymer, Polym.Test24(2005)613–619.[11]C_Y A.Wong,N.S.K.Ng,V.I.F.Ng,Colouring of mouldedplastic products by the addition of colour masterbatches, J.Mat.Proc.Tech.6(1997)468–471.[12]B.L.Kaul,Coloration of plastics using organic pigments,Rev.Prog.Coloration23(1993)19–35.[13]C-F.Ou,The crystallisation characteristics of polypropyleneand low ethylene content polypropylene copolymer with copolyester,Eur.Polym.J.38(2002)67–473.[14]S.Z.D.Cheng,J.J.Janimak, A.Q.Zhang, E.T.Hsieh,Isotacticity effect on crystallisation and melting in poly-propylene fractions.1:Crystalline-structures and thermodyn-amic property changes,Polymer32(6)(1991)648–655. [15]Y.Heng,X.Jin,J.N.Hay,Evaluation of multiple meltingpeaks of propylene-ethylene copolymers,Polym.J.30(3) (1998)215–221.[16]R.Paukkeri,A.Lehtinen,Thermal behaviour of polypropy-lene fractions:2.The multiple melting peaks,Polymer34(19) (1993)4083–4088.[17]C.Goetzloff,Borealis S.A,Austria,Private Communication toP Yayla2001.[18]B.Wunderlich,Macromolecular Physics Crystal Nucleation,Growth,Annealing,vol.2,Academic Press,New York,1976.p.46.[19]Y.Mubarak,P.J.Martin,E.Harkin-Jones,Effect of nucleatingagents and pigments on the crystallisation,morphology,and mechanical properties of polypropylene,pos.29(7)(2000)307–515.[20]R.A.Phillips,M.D.Wolkowitcz,in: E.P.Moore(Ed.),Structure and Morphology Polypropylene Handbook:Polym-erisation,Characterisation,Properties,Applications, Hanser/Gardner,Munich,1996,p.113.[21]S.Kening, A.Silberman,I.Dolgopolsky,The effects ofpigments on the crystallisation and properties of polypropy-lene,ANTEC’97(1997)2706–2710.[22]J.A.Krisher,S.S.Marshall,The Effects of colorant onmechanical properties of polypropylene,ANTEC’97(1997) 2928–2930.[23]The Effect of UV Light on Weather on Plastics andElastomers,Plastics Design Library,3rd ed.,William Andrew Inc,1997.[24]T.J.Turton,J.R.White,Effect of stabilizer and pigment onphoto-degradation depth profiles in polypropylene,Polym.Degrad.Stab.74(2001)559–568.[25]S.A.Hamid,A.G.Maadhah,M.B.Amin,in:S.H.Hamid,M.B.Amin,A.G.Maadhah(Eds.),Weathering Degradation in Polyethylene Handbook of Polymer Degradation,Marcel Dekker,New York,USA,1992.[26]A.J.Chirinos-Pedro´n,N.S.Allen,in:S.H.Hamid,M.B.Amin,A.G.Maadhah(Eds.),Aspects of Polymer StabilisationHandbook of Polymer Degradation,Marcel Dekker,New York,USA,1992.[27]W.L.Hawkins,Polymer degradation and stabilisation,Springer,Berlin,1984.[28]J.Fiebig,M.Gahleitner,C.Paulik,J.Wolfschwenger,Ageingof polypropylene:processes and consequences,Polym.Test.18(1999)257–266.[29]G.Romano,R.Russo,V.Vittoria,Influence of aging on themechanical properties of smectic polypropylene,J.Macromol.Sci.Phys.B37(6)(1998)841–850.S.Sahin,P.Yayla/Polymer Testing24(2005)1012–10211021。
effects of laser welding parameters on the heat input and weld-bead profile

Journal of Materials Processing Technology164–165(2005)978–985Effect of laser welding parameters on the heat input and weld-bead profileK.Y.Benyounis∗,A.G.Olabi,M.S.J.HashmiSchool of Mechanical and Manufacturing Engineering,Dublin City University,Dublin9,IrelandAbstractLaser butt-welding of medium carbon steel was investigated using CW1.5kW CO2laser.The effect of laser power(1.2–1.43kW),welding speed(30–70cm/min)and focal point position(−2.5to0mm)on the heat input and the weld-bead geometry(i.e.penetration(P),welded zone width(W)and heat affected zone width(W HAZ))was investigated using response surface methodology(RSM).The experimental plan was based on Box–Behnken design.Linear and quadratic polynomial equations for predicting the heat input and the weld-bead geometry were developed.The results indicate that the proposed models predict the responses adequately within the limits of welding parameters being used.It is suggested that regression equations can be used tofind optimum welding conditions for the desired criteria.©2005Elsevier B.V.All rights reserved.Keywords:Laser welding;RSM;Weld-bead profile1.IntroductionLaser welding has become an important industrial process because of its advantages as a bonding process over the other widely used joining ser welding characterize with parallel-sided fusion zone,narrow weld width and high penetration.These advantages came from its high power den-sity,which make the laser welding one of the keyhole welding processes[1].The laser welding input parameters determine the shape of laser weld-bead,due to the combination of these parameters control the heat input[2].For a good weld qual-ity the combination of the output power,welding speed,focal position,shielding gas and position accuracy should be cor-rectly selected[3].RSM is widely used to predict the weld-bead geometry and mechanical properties in many welding process[4–8].In this work RSM is used to develop models to predict the heat input and to describe the laser weld-bead profile(i.e.weld penetration,welded zone width and HAZ width)for CW CO2laser butt-welding of medium carbon steel.The laser input parameters taken into consideration are laser power(LP),welding speed(S)and focused position(F).∗Corresponding author.E-mail address:khaled.benyounis2@mail.dcu.ie(K.Y.Benyounis).2.Experimental designThe experiment was designed based on a three level Box–Behnken design with full replication[9].Laser power (1.2–1.43kW),welding speed(30–70cm/min)and focal point position(−2.5to0mm)being the laser independent input variables.Table1shows laser input variables and ex-perimental design levels used.RSM was applied to the ex-perimental data using statistical software,Design-expert V6. Linear and second order polynomials werefitted to the experi-mental data to obtain the regression equations.The sequential F-test,lack-of-fit test and other adequacy measures were used in selecting the best models.A step-wise regression method was used tofit the second order polynomial equation(1)to the experimental data and to identify the relevant model terms [10,11].The same statistical software was used to generate the statistical and response plots.Y=b0+b iχi+b iiχ2ii+b ijχiχj(1)3.Experimental workMedium carbon steel with chemical composition in weight percent of0.46%C,0.2%Si,0.7%Mn and Fe Balance was used as work piece material.The size of each plate was180mm long×80mm width with thickness of5mm.0924-0136/$–see front matter©2005Elsevier B.V.All rights reserved. doi:10.1016/j.jmatprotec.2005.02.060K.Y.Benyounis et al./Journal of Materials Processing Technology164–165(2005)978–985979 Table1Process variables and experimental design levels usedVariables−10+1Laser power,LP(kW)1.21.31251.425Welding speed,S(cm/min)305070Focused position,F(mm)−2.5−1.250Trial samples of butt-welding were performed by varyingone of the process variables to determine the working rangeof each variable.Absence of visible welding defects and atleast half depth penetration were the criteria of choosing theworking ranges.The experiment was carried out accordingto the design matrix in a random order to avoid any system-atic error using a CW1.5kW CO2Rofin laser provided byMechtronic Industries Ltd.Argon gas was used as shield-ing gas with constantflow rate of5l/min.Two transversespecimens were cut from each weldment.Standard metallo-graphic was made for each transverse specimen.The beadprofile parameters‘responses’were measured using an opti-cal microscope with digital micrometers attached to it withan accuracy of0.001mm,which allow to measure in X-axesand y-axes.The average of two measured weld profile parameters wasrecorded for each response.The design matrix and the aver-age measured responses are shown below in Tables2and3.4.Results and discussionThe results of the weld-bead profile were measured ac-cording to design matrix Table2using the transverse sec-tioned specimens and the optical microscope mentioned ear-lier,the measured responses are listed in Table3.Analysingthe measured responses by the design expert software.Thefit summary output indicates that the linear model is statis-tically significant for the penetration‘the second response’Table2Design matrix with code independent process variablesExp.No.Runorder Laserpower(kW)Welding speed(cm/min)Focusedposition(mm)11−1−10 281−10 313−110 414110 54−10−1 61610−1 710−101 83101 950−1−1 10701−1 1190−11 126011 1311000 1417000 152000 1615000 1712000Table3Experimental measured responsesExp.no.Heat input(J/cm)P(mm)W(mm)W HAZ(mm) 11920 3.572 2.3580.561 22280 4.322 2.8050.872 3823 2.705 1.3420.392 4977 3.651 1.8520.384 51152 2.655 2.7610.453 61368 3.888 3.3810.569 71152 3.813 2.0870.511 81368 4.539 2.5720.574 92100 3.905 3.6810.625 10900 2.367 1.9820.375 112100 4.987 2.4230.762 12900 3.824 1.6490.413 131260 3.712 2.6250.531 141260 3.872 2.2820.562 151260 3.586 2.5670.466 161260 3.505 2.4130.478 171260 3.626 2.2930.506 Table4ANOV A table for heat input reduced quadratic modelSource Sum ofsquaresd.f.Mean square F value Prob>FModel3246465481161611507<0.0001 LP111932.111119321587<0.0001 S28800001288000040833<0.0001 S2243952.912439523459<0.0001 LP×S10579.56110579150<0.0001 Residual846.37321270.53Correctedtotal324731116R2=0.9997;predicted R2=0.9989.Table5ANOV A table for penetration reduced linear modelSource Sum ofsquaresd.f.Mean square F value Prob>FModel 6.2793 2.09351.399<0.0001 LP 1.6701 1.67041.007<0.0001 S 2.2461 2.24655.158<0.0001 F 2.3631 2.36358.031<0.0001 Residual0.529130.041Lack-of-fit0.45190.0502.5600.190 Pure error0.07840.020Corrected total 6.80916R2=0.922,predicted R2=0.849;adjusted R2=0.904,adequate preci-sion=21.931.therefore it will be used for further analysis.While for the other responses the quadratic models are statistically recom-mended for further analysis.4.1.Analysis of variance(ANOVA)The test for significance of the regression models,the test for significance on individual model coefficients and the lack-of-fit test were performed using the same statistical package. By selecting the step-wise regression method,which elim-inates the insignificant model terms automatically,the re-980K.Y.Benyounis et al./Journal of Materials Processing Technology 164–165(2005)978–985Table 6ANOV A table for WZ width reduced quadratic model Source Sum of squares d.f.Mean square F value Prob >F Model 5.14060.85758.732<0.0001LP 0.53110.53136.4400.0001S 2.4661 2.466169.105<0.0001F 1.1811 1.18180.985<0.0001S 20.36110.36124.7500.001F 20.38610.38626.4480.0004S ×F 0.21410.21414.6660.003Residual 0.146100.015Lack-of-fit 0.04860.0080.3300.891Pure error 0.09840.024Corrected total5.28616R 2=0.972,predicted R 2=0.922;adjusted R 2=0.956,adequate preci-sion =29.498.Table 7ANOV A table for HAZ width reduced quadratic model Source Sum of squares d.f.Mean square F value Prob >F Model 0.25940.06542.631<0.0001LP 0.02910.02919.1380.0009S 0.19710.197129.953<0.0001F0.00710.0074.6660.0517LP ×S 0.02510.02516.7660.0015Residual 0.018120.002Lack-of-fit 0.01280.0020.9900.5436Pure error 0.00640.002Corrected total0.27716R 2=0.934,predicted R 2=0.861;adjusted R 2=0.912,adequate preci-sion =22.899.Fig.1.Scatter diagram of heatinput.Fig.2.Scatter diagram of penetration.sulting ANOV A Tables 4–7for the reduced quadratic mod-els summarise the analysis of variance of each response and show the significant model terms.The same tables show also the other adequacy measures R 2,adjusted R 2and predicted R 2.The entire adequacy measures are close to 1,which is in reasonable agreement and indicate adequate models.The ad-equate precision compares the range of the predicted value at the design points to the average prediction error.In all cases the value of adequate precision are dramatically greater than 4.The adequate precision ratio above 4indicates adequate model discrimination.The analysis of variance indicates that for the heat in-put model.The main effect of the laser power (LP),welding speed (S ),the second order effect of welding speed (S 2)and the two level interaction of laser welding and welding speed (LP ×S )are the most significant model terms associatedwithFig.3.Scatter diagram of WZ width.K.Y.Benyounis et al./Journal of Materials Processing Technology 164–165(2005)978–985981Fig.4.Scatter diagram of HAZ width.heat input.Secondly for the penetration model,the analysis indicated that there is a linear relationship between the main effects of the three parameters.Also,in case of welded zone width model the main effect of laser power (LP),welding speed (S ),focused position (F ),the second order effect of welding speed (S 2),the second order effect of the focused position (F 2)and the two level interaction of welding speed and focused position (SF)are significant model terms.However,the main effect of welding speed (S )and the main effect of focused position (F )are the most significant factors associated with the welded zone width.Finally,for HAZ width model it is evident that the main effect of laser power (LP),welding speed (S ),focused position (F )and the two level interaction of the laser power and welding speed (LP ×S )are significant model terms.However,the main ef-fect of welding speed (S )is the most important factorinfluentFig.5.3D graph show the effect of LP and S on the heatinput.Fig.6.Contours graph show the effect of LP and S on the heat input.the HAZ width.The final mathematical models in terms of coded factors as determined by design expert software are shown below:heat input =1260+118.29×LP −600×S +240×S 2−51.43×LP ×S(2)P =3.68+0.46×LP −0.53×S +0.54×F (3)W =2.42+0.26×LP −0.56×S −0.38×F −0.31×S 2+0.30×F 2+0.23×S ×F(4)Fig.7.3D graph shows the effect of LP and S on penetration at F =−1.25mm.982K.Y.Benyounis et al./Journal of Materials Processing Technology 164–165(2005)978–985Fig.8.Contour graph shows the effect of LP and S on penetration at F =−1.25mm.W HAZ =0.53+0.06×LP −0.16×S +0.03×F −0.08×LP ×S(5)While the following final empirical models in terms of actual factors:heat input =1380.002+2194.28×LP −60×S +0.6×S 2−22.86×LP ×S(6)Fig.9.Contour graph shows the effect of LP and S on the weld penetration at F =−2.5mm.Fig.10.Contour graph shows the effect of LP and S on the weld penetration at F =0mm.P =0.2162+4.061×LP −0.026494×S+0.43480×F(7)W =−1.78957+2.29111×LP +0.060984×S −0.28628×F −7.71842E −004×S 2+0.19345×F 2+9.25E −003×S ×F(8)Fig.11.3D graph shows the effect of S and F on the weld width at LP =1.31kW.K.Y.Benyounis et al./Journal of Materials Processing Technology 164–165(2005)978–985983Fig.12.Contour graph shows the effect of S and F on the weld width at LP =1.31kW.W HAZ =−2.0753+2.30778×LP +0.038671×S+0.0238×F −0.035444×LP ×S(9)4.2.Validation of the modelsFigs.1–4show the relationship between the actual and predicted values of heat input,P ,W and W HAZ ,respectively.These figures indicate that the developed models areadequateFig.13.Contour graph shows the effect of S and F on the weld width at LP =1.2kW.Fig.14.Contour graph shows the effect of S and F on the weld width at LP =1.41kW.because the residuals in perdiction of each response are min-imum,since the residuals tend to be close to the diagonal line.Furthermore,to verify the adequacy of the developed models,three confirmation experiments were carried out us-ing new test conditions,but are within the experiment range defined ing the point prediction option in the soft-ware,the heat input,P ,W and W HAZ of the validation exper-iments were predicted using the previous developed models.Table 8summarises the experiments condition,the actual ex-perimental values,the predicted values and the percentages oferror.Fig.15.3D graph shows the effect of LP and S on the HAZ width at F =−1.25mm.984K.Y.Benyounis et al./Journal of Materials Processing Technology 164–165(2005)978–985Fig.16.Contour graph shows the effect of LP and S on the HAZ width at F =−1.25mm.4.3.Effect of process factors on weld-bead parameters 4.3.1.Heat inputThe heat input is directly related to the laser power,the welding speed and welding efficiency.It can be calculated directly from heat input =(LP/S )×η,where ηis the welding efficiency.The welding efficiency is taking as 80%[12].The reason of predicting the heat input is to develop a model to include it into the optimisation step in future work.FromFig.17.Contour graph shows the effect of S and LP on the HAZ width at F =−2.5mm.Fig.18.Contour graph shows the effect of S and LP on the HAZ width at F =0mm.Figs.5and 6it is evident that as the LP increases and the S decreases the heat input increases.4.3.2.PenetrationFrom the results it is clear that the three parameters are significantly affecting the penetration (P ).These effects are due to the following:the increase in (LP)leads to an in-crease in the heat input,therefore,more molten metal and consequently more (P )will be achieved.However,the idea is reversed in the case of welding speed (S )effect,because the welding speed (S )matches an opposite with the heat ing a focused beam results in increasing the power den-sity,which mean the heat will localize in small metal portionTable 8Confirmation experimentsHeat inputP W W HAZ Exp.no.1a Actual 12964.0122.4280.573Predicted 1299.433.832.5050.551Error %−0.2644.75−3.07 3.99Exp.no.2b Actual 21004.4072.7030.714Predicted 21004.212.6660.688Error %04.681.39 3.78Exp.no.3c Actual 12603.9622.3980.579Predicted 12604.222.3370.561Error %−6.112.613.21aLaser power:1.35kW;welding speed:50cm/min;focused position:−1.25mm.b Laser power:1.31kW;welding speed:30cm/min;focused position:−1.25mm.c Laser power:1.31kW;welding speed:50cm/min;focused position:0mm.K.Y.Benyounis et al./Journal of Materials Processing Technology164–165(2005)978–985985resulting in increasing in the power density leading to bet-ter(P).To achieve maximum(P)the laser power has to be maximum with focused beam(i.e.F=0)while(S)has to be minimum.Figs.7–10show the effect of process parameters on the weld penetration.4.3.3.Welded zone widthThe results indicate that the welding speed(S)and fo-cused position(F)are the most important factors affecting the welded zone width(W).An increase in welding speed(S) leads to a decrease in(W).This is due to the laser beam travel-ling at high speed over the welding line when(S)is increased. Therefore the heat input decreases leading to less volume of the base metal being melted,consequently the width of the welded zone decreases.Moreover,defocused beam,which mean wide laser beam results in spreading the laser power onto wide area.Therefore,wide area of the base metal will melt leading to an increase in(W)or vice versa.The results show also that laser power(LP)contribute secondary effect in the WZ width dimensions.An increase in(LP)results in slightly increase in the(W),because of the increase in the power density.Figs.11–14show the effect of process param-eters on the WZ width.4.3.4.Heat affected zone widthThe main factor influencing the width of HAZ(W HAZ)is the welding speed as the results indicated.This is due to the fact that at low(S)the heat input will be greater.This heat will conduct from the fusion zone to the bulk metal through HAZ making it wider and coarser.The results show also that the other two factors and the two level interaction of the(LP×S) are contributing secondary effect in width of HAZ(W HAZ). Figs.15–18show the effect of process parameters on the HAZ width.5.ConclusionThe following conclusions were drawn from this investi-gation within the factors limits considered.1.Box–Behnken design can be employed to develop math-ematical models for predicting weld-bead geometry. 2.The desired high quality welds can be achieved by choos-ing the working condition using the developed models.3.Heat input plays an important rule in the weld-bead pa-rameters dimension.4.Welding speed has a negative effect on all the responsesinvestigated whereas;the laser power has a positive effect.5.As the focused position goes in the metal(F<0)the pen-etration significantly reduces and the HAZ width slightly reduces,but WZ width increases. AcknowledgementsLibyan Government is gratefully acknowledged for thefi-nancial support of this research.Technical support from Mr. Martin Johnson the laser welding expert and Mr.Michael May of Dublin City University are also gratefully acknowl-edged.References[1]W.M.Steen,Laser Material Processing,Springer,London,1991.[2]C.Dawes,Laser Welding,Abington Publishing,New York,NY,1992.[3]Q.Huang,J.Hagstroem,H.Skoog,G.Kullberg,Effect of laserparameter variation on sheet metal welding,Int.J.Join.Mater.3(3) (1991)79–88.[4]D.Kim,et al.,Modelling and optimisation of a GMA welding pro-cess by genetic algorithm and response surface methodology,Int.J.Prod.Res.40(7)(2002)1699–1711.[5]V.Gunaraj,N.Murugan,Application of response surface method-ology for predicting weld bead quality in SAW of pipes,J.Mater.Process.Technol.88(1999)266–275.[6]K.Y.Benyounis,A.H.Bettamer,A.G.Olabi,M.S.J.Hashmi,Pre-dicting the impact strength of spiral-welded pipe joints in SAW of low carbon steel,in:Proceedings of the IMC21,Limerick,Ireland, 2004.[7]V.Gunaraj,N.Murugan,Prediction of heat-affected zone character-istics in SAW of structural steel pipes,Weld.J.(2002)94s–98s. [8]T.T.Allen,R.W.Richardson,D.P.Tagliable,G.P.Maul,Statisticalprocess design for robotic GMA welding of sheet metal,Weld.J.(2002)69s–77s.[9]Design-Expert Software,Version6,User’s Guide,Technical Manual,Stat-Ease Inc.,Minneapolis,MN,2000.[10]D.C.Montgomery,Design and Analysis of Experiments,2nd ed.,Wiley,New York,1984.[11]A.I.Khuri,J.A.Cornell,Response Surfaces Design and Analysis,2nd ed.,Marcel Dekker,New York,1996.[12]P.W.Fuerschbach,Measurement and prediction of energy transferefficiency in laser welding,Weld.J.75(1996)24s–34s.。
机械设计制造专业毕业设计中英翻译--一残余应力针对不同的材料车削的建模方法
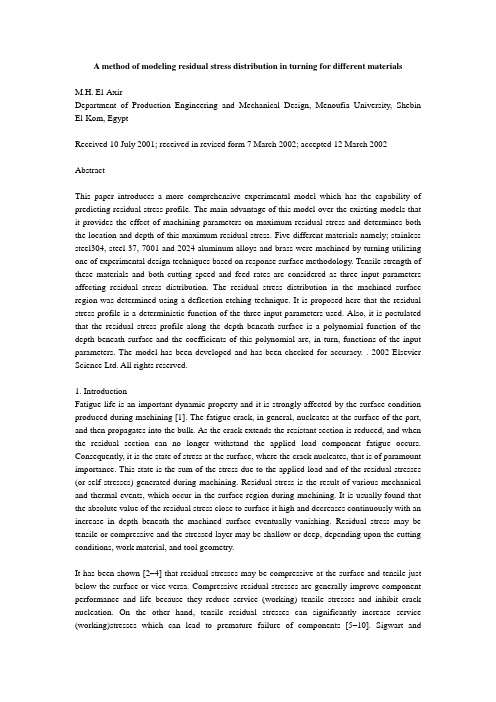
A method of modeling residual stress distribution in turning for different materialsM.H. El-AxirDepartment of Production Engineering and Mechanical Design, Menoufia University, Shebin El-Kom, EgyptReceived 10 July 2001; received in revised form 7 March 2002; accepted 12 March 2002 AbstractThis paper introduces a more comprehensive experimental model which has the capability of predicting residual stress profile. The main advantage of this model over the existing models that it provides the effect of machining parameters on maximum residual stress and determines both the location and depth of this maximum residual stress. Five different materials namely; stainless steel304, steel-37, 7001 and 2024-aluminum alloys and brass were machined by turning utilizing one of experimental design techniques based on response surface methodology. Tensile strength of these materials and both cutting speed and feed rates are considered as three input parameters affecting residual stress distribution. The residual stress distribution in the machined surface region was determined using a deflection-etching technique. It is proposed here that the residual stress profile is a deterministic function of the three input parameters used. Also, it is postulated that the residual stress profile along the depth beneath surface is a polynomial function of the depth beneath surface and the coefficients of this polynomial are, in turn, functions of the input parameters. The model has been developed and has been checked for accuracy. . 2002 Elsevier Science Ltd. All rights reserved.1. IntroductionFatigue life is an important dynamic property and it is strongly affected by the surface condition produced during machining [1]. The fatigue crack, in general, nucleates at the surface of the part, and then propagates into the bulk. As the crack extends the resistant section is reduced, and when the residual section can no longer withstand the applied load component fatigue occurs. Consequently, it is the state of stress at the surface, where the crack nucleates, that is of paramount importance. This state is the sum of the stress due to the applied load and of the residual stresses (or self stresses) generated during machining. Residual stress is the result of various mechanical and thermal events, which occur in the surface region during machining. It is usually found that the absolute value of the residual stress close to surface it high and decreases continuously with an increase in depth beneath the machined surface eventually vanishing. Residual stress may be tensile or compressive and the stressed layer may be shallow or deep, depending upon the cutting conditions, work material, and tool geometry.It has been shown [2–4] that residual stresses may be compressive at the surface and tensile just below the surface or vice versa. Compressive residual stresses are generally improve component performance and life because they reduce service (working) tensile stresses and inhibit crack nucleation. On the other hand, tensile residual stresses can significantly increase service (working)stresses which can lead to premature failure of components [5–10]. Sigwart andFessenmeyer [11], for example, reported that fatigue tests on turned specimens of 42CrMo4 steel presenting high tensile residual stresses (up to 600:800 MPa) showed a close to 30% reduction in the fatigue limit. Matsumoto et al. [12] reported that the fatigue strength of hardened AISI 4340 steel specimens (54 HRC) after flycutting was 2–5% higher that after grinding probably because the compressive residual stress distribution produced by the single point cutting operation penetrated to a grater work-piece depth. Similarly, Prata Pina et al. [13] found that, when milling annealed hot work die steel (AISI H13), residual stresses close to zero were obtained at the surface, dropping sharply to a maximum compressive stress approximately 100 μm below the surface, then rising again to the tensile side.Accordingly, It is very clear that the information concerning residual stresses profile (magnitude and direction along the depth) of the machined surface region will be valuable in the design and manufacture of parts. Therefore, it is important that the effect of the machining process parameters on the residual stress profile is determined, and subsequently, such machining parameters may be chosen which would enhance fatigue life by inducing favorable residual stress (compressive stress).The majority of the research existing in literature on the effect of machining parameters on the residual stress profile are experimental in nature. Very few analytical models are available. Liu and Barash [14,15] explained the formation of residual stress by considering the stress strain history that the surface layer experienced due to the movement of the cutting tool. Lin et al. [9] used finite element techniques to determine residual stress profiles in orthogonal machining. Wu and Matusmoto [16] also used finite element to determine factors, which affect residual stress formation in hardened steel machining. Devarajan et al. [17] constructed an experimental model for prediction of the surface residual stress. Although the surface residual stress is important, in most machining processes, the subsurface residual stresses are at least equally important.This paper introduces a more comprehensive experimental model to predict surface and subsurface residual stress profiles in turning of five different materials. With the help of this knowledge it will become possible to optimize machining parameters such that the surface integrity of the machined component for these five different materials is maximized under service conditions.2. Experimental details2.1. Workpiece materialsWorkpieces of stainless steel 304, steel37, aluminum alloy 7001, aluminum alloy 2024, and brass were utilized. These materials were selected because they have different machining characteristics and are important in industry. Moreover, both of aluminum alloys 7001 and 2024 are particularly well suited for parts and structures requiring high strength-to-weight ratios. The chemical compositions in weight percent and tensile strength are given in Table 1. The tool material employed was high-speed steel.2.2. Workpiece preparationThe five different materials were machined into ring shapes with the dimensions shown in Fig. 1a. Fig. 1b shows the tested ring mounted on its mandrel. It is probable that residual stresses areinduced in the surface region of the workpiece because of the machining involved in preparation, hence it was necessary to remove these stresses by annealing the workpieces.Stainless steel 304, steel 37, Al. 7001, Al. 2024 and free machining brass workpieces were heated to 800, 595, 340, 340, and 260°C for 3, 6, 2, 2 and 1 h, respectively, and then cooled in air or in furnace.In this investigation, the specimens were machined using one of the experimental designs. According to a central composed second-order rotatable design with three independent variables, the total number of experiments, N, was determined to be 20. The cutting conditions and their coded are summarized in Table 2.The residual stress distribution in the machined surface was determined utilizing a deflection etching technique where the residual stresses in the removal layer are relived and the remaining residual stresses are redistributed until a new equilibrium position is achieved. This change in shape can be measured from which residual stresses can be calculated. A layer of approximately 15–25 μm was removed with the help of electrochemical etching. Layers were removed until the residual stress state became negligible. The obtained residual stress profiles for 20 different combinations according to Table 3 are shown in Fig. 2.3. Proposed modelThe proposed model postulates that the residual stress profile as well as the depth of residual stress distribution are functions of the machining parameters. The model assumes that profile of residual stress along the depth is polynomial function of the depth. The profile can be represented as:where: si is the residual stress, cni are the coefficients of the nth order polynomial term and z is the depth beneath the machined surface.Further, it is proposed that the coefficients of the polynomial are individual functions of the machining parameters. The relation of the coefficient to the machining parameters iswhere Ci is the coefficient of polynomial for residual stress profile and bxi is the effect of factor (or interaction of factor) x.The values of the code number of each parameter, x, can be obtained from the following transformation equations.where V, F and T are cutting speed, feed and tensile strength of the material, respectively.The values of bxis are determined experimentally. The procedure is as follows:1. Twenty specimens are cut using different combinations (Table 3) of the five levels of each parameter used in this work.。
文献翻译-切削参数的改变对切削温度的影响
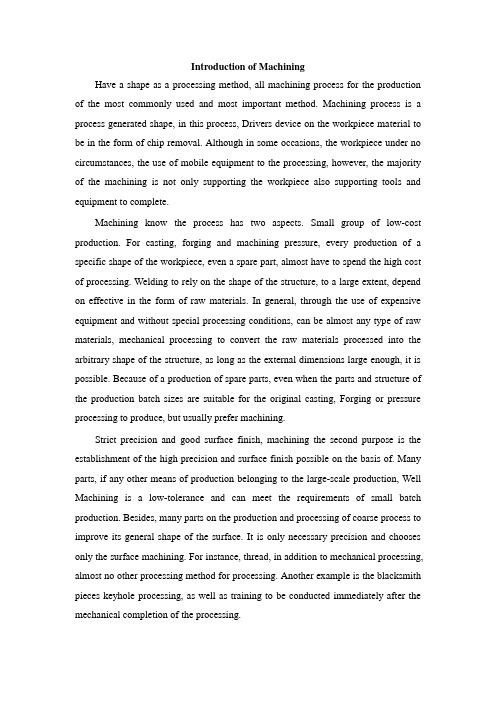
Introduction of MachiningHave a shape as a processing method, all machining process for the production of the most commonly used and most important method. Machining process is a process generated shape, in this process, Drivers device on the workpiece material to be in the form of chip removal. Although in some occasions, the workpiece under no circumstances, the use of mobile equipment to the processing, however, the majority of the machining is not only supporting the workpiece also supporting tools and equipment to complete.Machining know the process has two aspects. Small group of low-cost production. For casting, forging and machining pressure, every production of a specific shape of the workpiece, even a spare part, almost have to spend the high cost of processing. Welding to rely on the shape of the structure, to a large extent, depend on effective in the form of raw materials. In general, through the use of expensive equipment and without special processing conditions, can be almost any type of raw materials, mechanical processing to convert the raw materials processed into the arbitrary shape of the structure, as long as the external dimensions large enough, it is possible. Because of a production of spare parts, even when the parts and structure of the production batch sizes are suitable for the original casting, Forging or pressure processing to produce, but usually prefer machining.Strict precision and good surface finish, machining the second purpose is the establishment of the high precision and surface finish possible on the basis of. Many parts, if any other means of production belonging to the large-scale production, Well Machining is a low-tolerance and can meet the requirements of small batch production. Besides, many parts on the production and processing of coarse process to improve its general shape of the surface. It is only necessary precision and chooses only the surface machining. For instance, thread, in addition to mechanical processing, almost no other processing method for processing. Another example is the blacksmith pieces keyhole processing, as well as training to be conducted immediately after the mechanical completion of the processing.Primary Cutting ParametersCutting the work piece and tool based on the basic relationship between the following four elements to fully describe: the tool geometry, cutting speed, feed rate, depth and penetration of a cutting tool.Cutting Tools must be of a suitable material to manufacture, it must be strong, tough, hard and wear-resistant. Tool geometry -- to the tip plane and cutter angle characteristics -- for each cutting process must be correct.Cutting speed is the cutting edge of work piece surface rate; it is inches per minute to show. In order to effectively processing, and cutting speed must adapt to the level of specific parts -- with knives. Generally, the more hard work piece material, the lower the rate.Progressive Tool to speed is cut into the work piece speed. If the work piece or tool for rotating movement, feed rate per round over the number of inches to the measurement. When the work piece or tool for reciprocating movement and feed rate on each trip through the measurement of inches. Generally, in other conditions, feed rate and cutting speed is inversely proportional to。
随着人类社会的发展及人们对产品质量要求的进一步提高
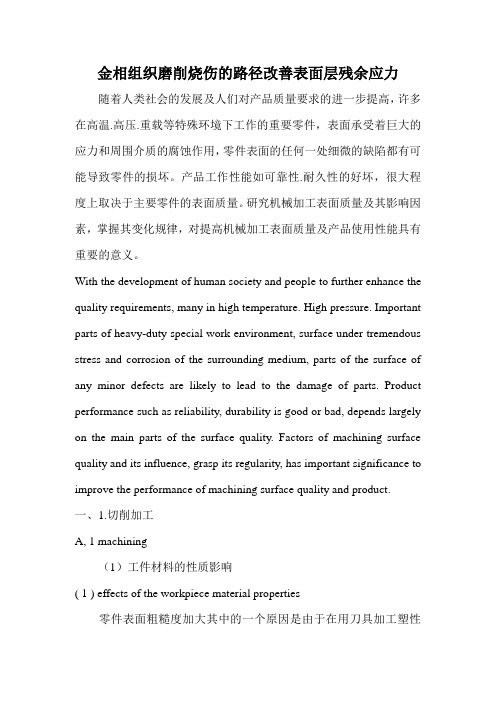
金相组织磨削烧伤的路径改善表面层残余应力随着人类社会的发展及人们对产品质量要求的进一步提高,许多在高温.高压.重载等特殊环境下工作的重要零件,表面承受着巨大的应力和周围介质的腐蚀作用,零件表面的任何一处细微的缺陷都有可能导致零件的损坏。
产品工作性能如可靠性.耐久性的好坏,很大程度上取决于主要零件的表面质量。
研究机械加工表面质量及其影响因素,掌握其变化规律,对提高机械加工表面质量及产品使用性能具有重要的意义。
With the development of human society and people to further enhance the quality requirements, many in high temperature. High pressure. Important parts of heavy-duty special work environment, surface under tremendous stress and corrosion of the surrounding medium, parts of the surface of any minor defects are likely to lead to the damage of parts. Product performance such as reliability, durability is good or bad, depends largely on the main parts of the surface quality. Factors of machining surface quality and its influence, grasp its regularity, has important significance to improve the performance of machining surface quality and product.一、1.切削加工A, 1 machining(1)工件材料的性质影响( 1 ) effects of the workpiece material properties零件表面粗糙度加大其中的一个原因是由于在用刀具加工塑性材料中,会发生塑性变形,再加上刀具在加工过程中会对零件有撕裂作用。
电主轴轴承座加工工艺流程

电主轴轴承座加工工艺流程1.首先准备加工设备和工具。
First, prepare the machining equipment and tools.2.对原材料进行切割和锻造处理。
Cut and forge the raw materials.3.清洁和除锈原材料表面。
Clean and remove rust from the surface of the raw materials.4.进行车削和铣削操作,精确加工轴承座的外形和尺寸。
Perform turning and milling operations to accurately machine the shape and dimensions of the bearing seat.5.对轴承座进行螺纹加工。
Thread machining on the bearing seat.6.进行热处理,增强轴承座的硬度和耐磨性。
Perform heat treatment to enhance the hardness and wear resistance of the bearing seat.7.进行表面抛丸处理,增加轴承座表面的粗糙度。
Perform surface shot blasting to increase the roughness of the bearing seat surface.8.进行喷漆或电镀处理,提高轴承座的防锈和美观性能。
Perform painting or plating treatment to improve the anti-rust and aesthetics of the bearing seat.9.进行装配和整体检查,确保轴承座的质量合格。
Assemble and inspect the overall quality of the bearing seat to ensure it meets standards.10.对成品进行包装和标识,准备出厂。
激光送粉增材制造 选区熔化精度
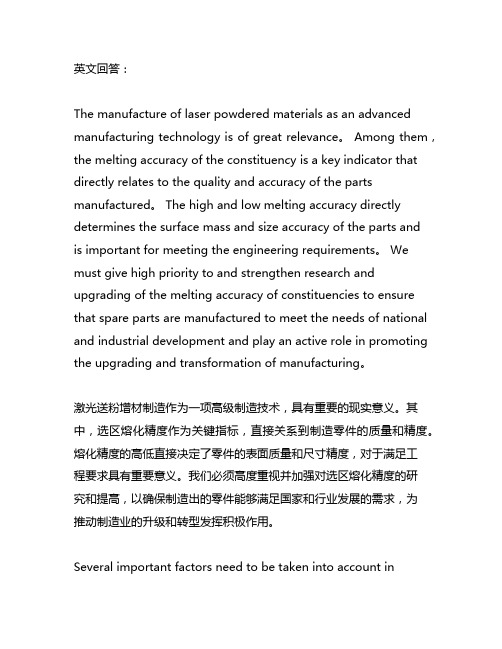
英文回答:The manufacture of laser powdered materials as an advanced manufacturing technology is of great relevance。
Among them,the melting accuracy of the constituency is a key indicator that directly relates to the quality and accuracy of the parts manufactured。
The high and low melting accuracy directly determines the surface mass and size accuracy of the parts andis important for meeting the engineering requirements。
We must give high priority to and strengthen research and upgrading of the melting accuracy of constituencies to ensure that spare parts are manufactured to meet the needs of national and industrial development and play an active role in promoting the upgrading and transformation of manufacturing。
激光送粉增材制造作为一项高级制造技术,具有重要的现实意义。
其中,选区熔化精度作为关键指标,直接关系到制造零件的质量和精度。
熔化精度的高低直接决定了零件的表面质量和尺寸精度,对于满足工程要求具有重要意义。
固定球阀主阀体关键形位公差评价及加工
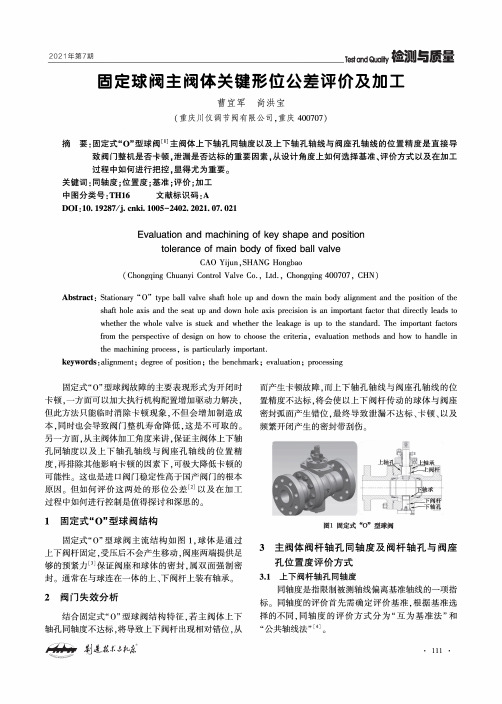
shaft hole axis and the seat up and down hole axis precision is an important factor that directly leads to whether the whole valve is stuck and whether the leakage is up to the standard. The important factors from the perspective of design on how to choose the criteria, evaluation methods and how to handle in the machining process, is particularly important. keywords: alignment ; degree of position ; the benchmark ; evaluation ; processing
3.1.5主阀体轴孔同轴度评价方式选择 “互为基准法”结合主阀体结构,存在两个问题:
(1)两轴孔轴线较短,轴线方向测量不稳定。(2)基准 圆柱和被测圆柱相距越远 ,基准圆柱轴向误差被线性 放大的越大。通过对图3分析,当“C”越大,基准轴线 延伸后与被评测轴线的距离“D”就越大。而“公共轴 线法”的基准轴是被测圆柱面和基准圆柱面截面圆心 通过“最小二乘法”产生的,如图4。这条公共轴线近 似于一个模拟心轴,更接近零件的实际装配过程。因 此,对主阀体上下轴孔同轴度的评价宜采用“公共轴 线法”。 3.2上下轴孔与阀座孔位置精度评价方式
工艺参数对AA7075热冲压摩擦性能的影响外文文献翻译、中英文翻译
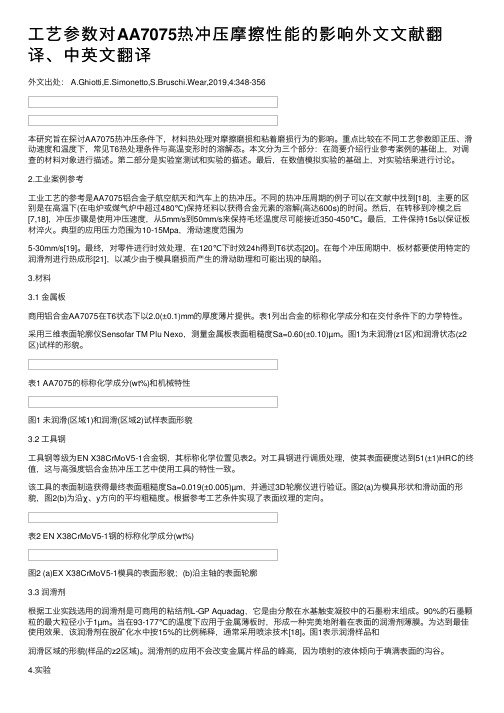
⼯艺参数对AA7075热冲压摩擦性能的影响外⽂⽂献翻译、中英⽂翻译外⽂出处: A.Ghiotti,E.Simonetto,S.Bruschi.Wear,2019,4:348-356本研究旨在探讨AA7075热冲压条件下,材料热处理对摩擦磨损和粘着磨损⾏为的影响。
重点⽐较在不同⼯艺参数即正压、滑动速度和温度下,常见T6热处理条件与⾼温变形时的溶解态。
本⽂分为三个部分:在简要介绍⾏业参考案例的基础上,对调查的材料对象进⾏描述。
第⼆部分是实验室测试和实验的描述。
最后,在数值模拟实验的基础上,对实验结果进⾏讨论。
2.⼯业案例参考⼯业⼯艺的参考是AA7075铝合⾦⼦航空航天和汽车上的热冲压。
不同的热冲压周期的例⼦可以在⽂献中找到[18],主要的区别是在⾼温下(在电炉或煤⽓炉中超过480℃)保持坯料以获得合⾦元素的溶解(⾼达600s)的时间。
然后,在转移到冷模之后[7,18],冲压步骤是使⽤冲压速度,从5mm/s到50mm/s来保持⽑坯温度尽可能接近350-450℃。
最后,⼯件保持15s以保证板材淬⽕。
典型的应⽤压⼒范围为10-15Mpa,滑动速度范围为5-30mm/s[19]。
最终,对零件进⾏时效处理,在120℃下时效24h得到T6状态[20]。
在每个冲压周期中,板材都要使⽤特定的润滑剂进⾏热成形[21],以减少由于模具磨损⽽产⽣的滑动助理和可能出现的缺陷。
3.材料3.1 ⾦属板商⽤铝合⾦AA7075在T6状态下以2.0(±0.1)mm的厚度薄⽚提供。
表1列出合⾦的标称化学成分和在交付条件下的⼒学特性。
采⽤三维表⾯轮廓仪Sensofar TM Plu Nexo,测量⾦属板表⾯粗糙度Sa=0.60(±0.10)µm。
图1为未润滑(z1区)和润滑状态(z2区)试样的形貌。
表1 AA7075的标称化学成分(wt%)和机械特性图1 未润滑(区域1)和润滑(区域2)试样表⾯形貌3.2 ⼯具钢⼯具钢等级为EN X38CrMoV5-1合⾦钢,其标称化学位置见表2。
可机加工性英文翻译
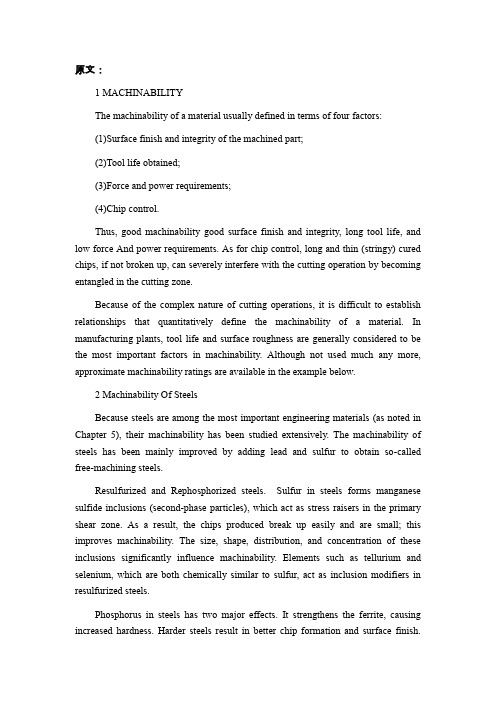
原文:1 MACHINABILITYThe machinability of a material usually defined in terms of four factors:(1)Surface finish and integrity of the machined part;(2)Tool life obtained;(3)Force and power requirements;(4)Chip control.Thus, good machinability good surface finish and integrity, long tool life, and low force And power requirements. As for chip control, long and thin (stringy) cured chips, if not broken up, can severely interfere with the cutting operation by becoming entangled in the cutting zone.Because of the complex nature of cutting operations, it is difficult to establish relationships that quantitatively define the machinability of a material. In manufacturing plants, tool life and surface roughness are generally considered to be the most important factors in machinability. Although not used much any more, approximate machinability ratings are available in the example below.2 Machinability Of SteelsBecause steels are among the most important engineering materials (as noted in Chapter 5), their machinability has been studied extensively. The machinability of steels has been mainly improved by adding lead and sulfur to obtain so-called free-machining steels.Resulfurized and Rephosphorized steels. Sulfur in steels forms manganese sulfide inclusions (second-phase particles), which act as stress raisers in the primary shear zone. As a result, the chips produced break up easily and are small; this improves machinability. The size, shape, distribution, and concentration of these inclusions significantly influence machinability. Elements such as tellurium and selenium, which are both chemically similar to sulfur, act as inclusion modifiers in resulfurized steels.Phosphorus in steels has two major effects. It strengthens the ferrite, causing increased hardness. Harder steels result in better chip formation and surface finish.Note that soft steels can be difficult to machine, with built-up edge formation and poor surface finish. The second effect is that increased hardness causes the formation of short chips instead of continuous stringy ones, thereby improving machinability.Leaded Steels. A high percentage of lead in steels solidifies at the tip of manganese sulfide inclusions. In non-resulfurized grades of steel, lead takes the form of dispersed fine particles. Lead is insoluble in iron, copper, and aluminum and their alloys. Because of its low shear strength, therefore, lead acts as a solid lubricant (Section 32.11) and is smeared over the tool-chip interface during cutting. This behavior has been verified by the presence of high concentrations of lead on the tool-side face of chips when machining leaded steels.Calcium-Deoxidized Steels. An important development is calcium-deoxidized steels, in which oxide flakes of calcium silicates (CaSo) are formed. These flakes, in turn, reduce the strength of the secondary shear zone, decreasing tool-chip interface and wear. Temperature is correspondingly reduced. Consequently, these steels produce less crater wear, especially at high cutting speeds.Stainless Steels. Austenitic (300 series) steels are generally difficult to machine. Chatter can be s problem, necessitating machine tools with high stiffness. However, ferritic stainless steels (also 300 series) have good machinability. Martensitic (400 series) steels are abrasive, tend to form a built-up edge, and require tool materials with high hot hardness and crater-wear resistance. Precipitation-hardening stainless steels are strong and abrasive, requiring hard and abrasion-resistant tool materials.The Effects of Other Elements in Steels on Machinability. The presence of aluminum and silicon in steels is always harmful because these elements combine with oxygen to form aluminum oxide and silicates, which are hard and abrasive. These compounds increase tool wear and reduce machinability. It is essential to produce and use clean steels.Carbon and manganese have various effects on the machinability of steels, depending on their composition. Plain low-carbon steels (less than 0.15% C) can produce poor surface finish by forming a built-up edge. Cast steels are more abrasive, although their machinability is similar to that of wrought steels. Tool and die steels are very difficult to machine and usually require annealing prior to machining. Machinability of most steels is improved by cold working, which hardens the materialand reduces the tendency for built-up edge formation.Other alloying elements, such as nickel, chromium, molybdenum, and vanadium, which improve the properties of steels, generally reduce machinability. The effect of boron is negligible. Gaseous elements such as hydrogen and nitrogen can have particularly detrimental effects on the properties of steel. Oxygen has been shown to have a strong effect on the aspect ratio of the manganese sulfide inclusions; the higher the oxygen content, the lower the aspect ratio and the higher the machinability.In selecting various elements to improve machinability, we should consider the possible detrimental effects of these elements on the properties and strength of the machined part in service.Sulfur can severely reduce the hot workability of steels, because of the formation of iron sulfide, unless sufficient manganese is present to prevent such formation. At room temperature, the mechanical properties of resulfurized steels depend on the orientation of the deformed manganese sulfide inclusions (anisotropy). Rephosphorized steels are significantly less ductile, and are produced solely to improve machinability.3 Machinability of Various Other MetalsAluminum is generally very easy to machine, although the softer grades tend to form a built-up edge, resulting in poor surface finish. High cutting speeds, high rake angles, and high relief angles are recommended. Wrought aluminum alloys with high silicon content and cast aluminum alloys may be abrasive; they require harder tool materials. Dimensional tolerance control may be a problem in machining aluminum, since it has a high thermal coefficient of expansion and a relatively low elastic modulus.Beryllium is similar to cast irons. Because it is more abrasive and toxic, though, it requires machining in a controlled environment.Cast gray irons are generally machinable but are. Free carbides in castings reduce their machinability and cause tool chipping or fracture, necessitating tools with high toughness. Nodular and malleable irons are machinable with hard tool materials.Cobalt-based alloys are abrasive and highly work-hardening. They require sharp, abrasion-resistant tool materials and low feeds and speeds.4 Machinability of Various MaterialsGraphite is abrasive; it requires hard, abrasion-resistant, sharp tools.Thermoplastics generally have low thermal conductivity, low elastic modulus, and low softening temperature. Consequently, machining them requires tools with positive rake angles (to reduce cutting forces), large relief angles, small depths of cut and feed, relatively high speeds, andproper support of the workpiece. Tools should be sharp.External cooling of the cutting zone may be necessary to keep the chips from bec oming “gummy” and sticking to the tools. Cooling can usually be achieved with a jet of air, vapor mist, or water-soluble oils. Residual stresses may develop during machining. To relieve these stresses, machined parts can be annealed for a period of time at temperatures ranging from C ︒80 to C ︒160 (F ︒175to F ︒315), and then cooled slowly and uniformly to room temperature.Thermosetting plastics are brittle and sensitive to thermal gradients during cutting. Their machinability is generally similar to that of thermoplastics.Because of the fibers present, reinforced plastics are very abrasive and are difficult to machine. Fiber tearing, pulling, and edge delamination are significant problems; they can lead to severe reduction in the load-carrying capacity of the component. Furthermore, machining of these materials requires careful removal of machining debris to avoid contact with and inhaling of the fibers.译文:1 可机加工性一种材料的可机加工性通常以四种因素的方式定义:(1)分的表面光洁性和表面完整性。
- 1、下载文档前请自行甄别文档内容的完整性,平台不提供额外的编辑、内容补充、找答案等附加服务。
- 2、"仅部分预览"的文档,不可在线预览部分如存在完整性等问题,可反馈申请退款(可完整预览的文档不适用该条件!)。
- 3、如文档侵犯您的权益,请联系客服反馈,我们会尽快为您处理(人工客服工作时间:9:00-18:30)。
Journal of Materials Processing Technology 139(2003)628–634Effect of machining parameters and heat treatment on the residual stress distribution in titanium alloy IMI-834B.R.Sridhar ∗,G.Devananda,K.Ramachandra,Ramaraja BhatGas Turbine Research Establishment,Bangalore 560093,IndiaAbstractThe residual stress variation in titanium alloy IMI-834as a function of depth following milling at different feeds,speeds and depths of cut was determined by a strain-gauge technique involving blind-hole drilling.The residual stresses were found to be compressive in nature and to be dependent upon the milling parameters.Heat treatment was found to relieve the residual stresses,the degree of stress relief being found to increase with increase in temperature.Optimum temperatures were determined at which significant relaxation occurred without adversely affecting the microstructure and mechanical behaviour of the material.©2003Elsevier B.V .All rights reserved.Keywords:IMI-834;Strain-gauge technique;Residual stress1.IntroductionIMI-834is a near-␣titanium alloy with the nominal com-position Ti–5.8Al–4.5Sn–4Zr–0.7Nb–0.5Mo–0.40Si–0.06C.This is a creep resistant alloy with a service temperature up to 600◦C and is primarily intended for gas turbine aero-engine applications such as discs,rings and blades.Residual stresses play an important role in determining the fatigue life of a component.This calls for relieving these stresses in such cases where an adverse effect is seen on the fatigue life.This paper highlights the variation in the magnitude and distribution of residual stresses locked up in the material following a milling operation at different speeds,feeds and depths of cut.The effects of varying temperature in relieving these stresses have also been discussed in the paper.Residual stresses were measured by the standard strain-gauge technique involving blind-hole drilling [1–7].The method calls for the use of a strain-gauge rosette of the size 25mm ×25mm (shown schematically in Fig.1)supplied by Micro-measurement Group,USA [5].If ε1,ε2and ε3are the values of the relieved strain noted from strain gauges 1,2and 3,respectively,of the strain gauge rosette used during blind-hole drilling,the principal residual stresses σmax and σmin are calculated as below:∗Corresponding author.σmax=ε3+ε14A −(ε3−ε1)2+(ε3+ε1−2ε2)24B(1)σmin =ε3+ε14A+(ε3−ε1)2+(ε3+ε1−2ε2)24B(2)tan 2α=ε3+ε1−2ε2ε3−ε1(3)whereA =−(1+ν)¯a 2Eand B =−¯b2E(4)in which αis the angle made by σmax with respect to gauge1,νthe Poisson’s ratio of the material and ¯a and ¯bare the uniform stress coefficients determined by Schajer by finite element studies,and available as a function of Z /D (hole depth/hole diameter).The residual stresses in the cutting direction (σc )and nor-mal direction (σn )were calculated using the expressions:σc +σn =σmax +σmin(5)σc −σn =(σmax −σmin )cos 2θ(6)where θis the angle made by σc with σmax .2.Experimental proceduresHot-rolled,solution-treated and ground pickled 50mmB.R.Sridhar et al./Journal of Materials Processing Technology139(2003)628–634629Fig.1.Strain-gauge rosette arrangement for measuring residual stresses. rectangular test-pieces.In order to remove the residual stresses induced during the fabrication of specimens,the test-pieces were stress relieved by keeping them at600◦C for1h in a vacuum furnace followed by an argon gas quench.The machining operations were carried out on FN-2V HMT milling lathe,5HP capacity,using a T Max K-20face milling cutter of50mm diameter and four TN-35-M titanium nitride coated inserts(TPAN-1603PPN,WIDIA).Servo Cut ‘S’1:20soluble oil was employed as a cuttingfluid.The test-pieces were milled at different milling parameters by varying the speed(v,m/min),feed(f,mm/tooth/rev.)and depth of cut(d,mm)as given in Table1.Test-pieces milled with the parameters,v=11m/min, f=0.056mm/tooth/rev.,d=0.25mm were stress relieved at400,500and600◦C for1h in a vacuum furnace followed by an argon gas quench.Both the machined and heat-treated test-pieces were sub-jected to residual stress measurement by blind-hole drilling.Table1Milling parametersSl.no.v(m/min)f(mm/tooth/rev.)d(mm) 111.000.0560.250 211.000.1000.250 356.000.0560.250 456.000.1000.250 511.000.056 2.000 611.000.100 2.000 756.000.056 2.000 856.000.100 2.000 935.000.075 1.125An air turbine assembly was used to drill a blind hole at the centre of the strain-gauge rosette(Fig.1)used in the exper-iment.Drilling was carried out at incremental depths and at each depth the relieved strains,ε1,ε2andε3(of the strain gauges1,2and3from the strain-gauge rosette)were noted. These strains were used in the evaluation of residual stresses by the use of Eqs.(1)–(6).3.ResultsFigs.2–9present plots of the residual stress distribution as functions of depth below the surface for different milling parameters employed in the present study.Negative values of the maximum and minimum residual stresses indicate that the residual stresses were compressive in nature.The maximum stresses in the cutting and normal directions were found to be at a depth of about0.1mm below the surface.3.1.Effect of feed(constant v and d)At low speed(11m/min)the residual stresses were found to decrease with increase of feed(cf.Figs.2and3).However, at high speed(56m/min)they were found to increase with increase in feed(cf.Figs.4and5;Figs.8and9).The peak residual stress was also observed to slightly shift towards the surface with decrease in feed(cf.Figs.2and3;Figs.4 and5;Figs.6and7).3.2.Effect of cutting velocity(constant f and d)The magnitude of the compressive stresses increased with increase in cutting velocity(cf.Figs.3and5;Figs.7and9). However,the magnitude was found to decrease with increase in cutting velocity at low values of feed and depth of cut (cf.Figs.2and4).Also,with increase in cutting speed,the peak residual stress was found to shift to the surface(Figs.3 and5;Figs.6and8).Further,in a few cases the depth of the residually stressed layer increased with increase in the magnitude of the residual stress.3.3.Effect of depth of cut(constant v and f)The magnitude of the residual stresses decreased with increase in depth of cut(cf.Figs.2and6;Figs.3and7; Figs.4and8;Figs.5and9).The peak residual stress was found to shift slightly towards the surface with increase in depth of cut.No obvious trend was seen pertaining to the depth of the residually stressed layer.3.4.Effect of heat treatmentFigs.10–12reveal the effect of heat treatment at different temperatures(400–600◦C)on the magnitude and distribu-tion of the residual stresses.It was found that the magnitude630 B.R.Sridhar et al./Journal of Materials Processing Technology 139(2003)628–634Fig.2.Variation of residual stresses due to vertical face milling as a function of depth.IMI-834;cutting speed,11m/min;feed,0.056mm/tooth/rev.;depth of cut,0.25mm.with increase in temperature.The degree of relaxation was 60%at 400◦C,75%at 500◦C and 90%at 600◦C.The re-laxation rate was very high in the initial 0.25mm of depth but thereafter it decreased,the degree of relaxation being almost linear with depth (Figs.13and 14).4.DiscussionThe results obtained for IMI-834subsequent to a milling operation were consistent with those obtained for titanium alloys,IMI-318(Ti–6Al–4V)and IMI-685(Ti–6Al–5Zr–0.5Mo–0.25Si)in the machined and polished condition [6].Shift of the peak residual stress to the surface indicated an increase in the degree of cold work [8,9].How-Fig.3.Variation of residual stresses due to vertical face milling as a func-tion of depth.IMI-834;cutting speed,11m/min;feed,0.1mm/tooth/rev.;ever,this is contradicted by a decrease in the peak residual stress,which is also supposed to increase with increase in the level of cold work in the surface layers [8,9].These indicate that the milling parameters such as speed,feed and depth of cut have a role to play in determining the extent of cold work during a machining operation.The effect of the machining parameters on the resulting residual stress and other response variables has been studied theoretically by different authors [10,11].Accordingly,the dependence of the residual stresses on the milling parameters could be explained by the following linear equation:σ=b 0+b 1v +b 2f +b 3d(7)In Eq.(7),coefficients b 0,b 1,b 2and b 3can be determined by experimental data relating the residual stresses to the milling parameters.As per this equation the residual stressesvaryFig.4.Variation of residual stresses due to vertical face milling as a function of depth.IMI-834;speed,56m/min;feed,0.056mm/tooth/rev.;B.R.Sridhar et al./Journal of Materials Processing Technology 139(2003)628–634631Fig.5.Variation of residual stresses due to vertical face milling as a function of depth.IMI-834;cutting speed,56m/min;feed,0.1mm/tooth/rev.;depth of cut,0.25mm.linearly with respect to any parameter provided that there is constancy of the other two.For example,at the same f and d the magnitude of residual stress increases or decreases,respectively,with increase or decrease of v .However,as ex-plained earlier and as per the residual stress data presented in Table 2,this trend was not shown consistently.This in-dicated that the milling parameters,in addition to acting in-dependently,are likely to interact with each other in deter-mining the residual stress state of the material.Adding some interactive terms [10,11],Eq.(7)could be modified as:σ=b 0+b 1v +b 2f +b 3d +b 4v f +b 5v d +b 6fd +b 7v fd(8)Fig.6.Variation of residual stresses for vertical face milling as a function of depth.IMI-834;cutting speed,11m/min;feed,0.056/tooth/rev.;depth Eq.(8)was solved using the generated data for the residual stresses in the cutting (σct )and normal (σnt )directions and relationships were obtained as given below:σct =−1784.2+24.9v +17392.6f +438.1d −393v f−3.4v d −4593.8fd +69.7v fd(9)σnt =−1782.5+18.5v +16221.1f +389.8d−293.9v f −v d −3797fd +38.6v fd(10)The residual stresses in the cutting and normal directions were evaluated using Eqs.(9)and (10),beinghighlightedFig.7.Variation of residual stresses due to vertical face milling as a func-tion of depth.IMI-834;cutting speed,11m/min;feed,0.1mm/tooth/rev.;632 B.R.Sridhar et al./Journal of Materials Processing Technology 139(2003)628–634Fig.8.Variation of residual stresses due to vertical face milling as a func-tion of depth.IMI-834;cutting speed,56m/min;feed,0.056mm/tooth/rev.;depth of cut,2.0mm.in Tables 3and 4.Experimental and calculated values were found to match reasonably with minimal errors.Residual stress relaxation in titanium was found to start at about 280◦C [12].Stress relaxations have been associ-ated with the onset of micro-plastic deformations resulting in the rearrangement/annihilation of dislocations [13].Any processes such as precipitations can adversely affect these phenomena and reduce the relaxation rates.Relaxations observed at 400,500and 600◦C in IMI-834were consistent with the generally observed trend.Titanium alloy IMI-834is similar to titanium alloy IMI-685that was found to be prone to silicide precipitation [14].The increase in stress relaxation with increase in temperature indicated that no deleterious precipitations such as silicidesoccurredFig.9.Variation of residual stresses due to vertical face milling as a func-tion of depth.IMI-834;cutting speed,56m/min;feed,0.1mm/tooth/rev.;Fig.10.Variation of residual stresses for vertical face milling:cutting speed,11m/min;feed,0.056/tooth/rev.;depth of cut,0.25mm.Stress relieved at 400◦C.Fig.11.Variation of residual stresses for vertical face milling:cutting speed,11m/min;feed,0.056mm/tooth/rev.;depth of cut,0.25mm.Stress relieved at 500◦C.Fig.12.Variation of residual stresses for vertical face milling:cutting speed,11m/min;feed,0.056mm/tooth/rev.;depth of cut,0.25mm.StressB.R.Sridhar et al./Journal of Materials Processing Technology 139(2003)628–634633Fig.13.Variation of residual stress in the cutting direction,σc for vertical face milling as a function of depth at different temperatures cutting speed,11m/min;feed,0.056mm/tooth/rev.;depth of cut,0.25mm.Table 2Peak residual stresses for different milling parameters Sl.no.v (m/min)f (mm/tooth/rev.)d (mm)σc (MPa)σn (MPa)1a 11.000.0560.250−738.72−806.042a 56.000.0560.250−604.02−701.043b 11.000.100 2.000−174.31−198.564b 56.000.100 2.000−502.44−429.741c 11.000.0560.250−738.72−806.045c 11.000.1000.250−205.93−271.828d 56.000.056 2.000−238.17−274.534d 56.000.100 2.000−502.44−429.741e 11.000.0560.250−738.72−806.047e 11.000.056 2.000−412.49−473.096f 56.000.1000.250−815.57−730.304f56.000.1002.000−502.44−429.74a With increase in v at low f and d ,the peak residual stresses have decreased.bWith increase in v at high f and d ,the peak residual stresses have increased.c With increase in f at low v and d ,the peak residual stresses have decreased.d With increase in f at high v and d ,the peak residual stresses have increased.e With increase in d at low v and f ,the peak residual stresses have decreased.f With increase in d at high v and f ,the peak residual stresses have decreased.Table 3Peak residual stresses (σc )in the cutting direction a ,b Sl.no.v (m/min)f (mm/tooth/rev.)d (mm)σc (MPa)σct (MPa)(σc −σct )(MPa)(σc −σct )2(MPa)2111.000.0560.250−738.72−732.12−6.6043.56211.000.1000.250−205.93−199.15−6.7845.97356.000.0560.250−604.02−597.58−6.4441.47456.000.1000.250−815.57−808.19−7.3754.32511.000.056 2.000−412.49−405.93−6.5643.03611.000.100 2.000−174.31−167.61−6.7044.89756.000.056 2.000−238.17−231.79−6.3740.58856.000.100 2.000−502.44−495.43−7.0049.00935.000.075 1.125−445.93−463.61−17.68312.581035.000.0751.125−410.81−463.61−52.802787.84a Sum of squared error =3463.24.Fig.14.Variation of the relaxation of residual stress in the cutting direction,σc as a function of depth at different temperatures.634 B.R.Sridhar et al./Journal of Materials Processing Technology139(2003)628–634Table4Peak residual stresses(σn)in the normal direction a,bSl.no.v(m/min)f(mm/tooth/rev.)d(mm)σnσnt(MPa)(σn−σnt)(MPa)(σn−σnt)2(MPa)2 111.000.0560.250−806.04−804.18−1.85 3.42211.000.1000.250−271.82−269.82−1.99 3.96356.000.0560.250−701.04−699.30−1.73 2.99456.000.1000.250−730.30−727.86−2.44 5.95511.000.056 2.000−473.09−471.28−1.81 3.28611.000.100 2.000−198.56−196.63−1.93 3.72756.000.056 2.000−274.53−272.94−1.59 2.53856.000.100 2.000−429.74−427.60−2.14 4.58935.000.075 1.125−471.91−496.4624.55602.701035.000.075 1.125−480.70−496.4615.76248.38a Sum of squared error=881.51.bσnt=−1782.5+18.5v+16221.1f+389.8d−293.9v f−v d−3797fd+38.6v fd.at the stress relieving temperatures employed.Greater re-laxation at the surface compared to the core(Fig.14) could be attributed to the effect of greater residual stress gradients at the surface than at the core[11,15].Major contribution to the overall stress relaxation appeared to originate from the initial200to300m of the surface layers.5.Conclusions1.Residual stresses due to the milling operations forthe milling parameters employed were compressive in nature.2.The variation in the magnitudes of the residual stressesdid not reveal any definite trend with respect to the milling parameters.3.A linear relationship could not explain the variation ofthe residual stresses with respect to the milling parame-ters,but a polynomial relationship involving both linear and interactive terms could explain the variations with reasonable accuracy.4.Stress relieving treatments led to the relaxation ofresidual stresses almost linearly with depth from the surface.5.The relaxation rates increased with increasing tempera-ture and did not reveal any evidence for deleterious pre-cipitation such as of silicides.6.The greater relaxation rates at the surface compared tothe core could be attributed to the steeper residual stress gradients at the surface than at the core.7.The major contribution to the overall stress relaxationappeared to originate from the surface layers.AcknowledgementsThe authors express their sincere thanks to the Director, GTRE,for extending facilities and giving encouragement throughout the course of the present work.References[1]J.Mathar,Trans.ASME56(4)(1934)249–254.[2]R.A.Kelsey,Proc.Soc.Exp.Stress Anal.14(1)(1956)181–194.[3]R.J.Rendler,I.Vigness,Proc.Soc.Exp.Stress Anal.23(2)(1966)577–586.[4]G.S.Schajer,J.Eng.Mater.Technol.110(4)(1988)338–349.[5]Technical Bulletin304-F and Instruction Manual for RS-200-01Milling Guide,Measurements Group Inc.,Raleigh,USA,1988. [6]B.R.Sridhar,W.G.Nafde,K.A.Padmanabhan,J.Mater.Sci.27(1992)5783–5788.[7]Annual Book of ASTM Standards,E837-45,ASTM,Philadelphia,1987,pp.991–997.[8]B.R.Sridhar,K.Ramachandra,K.A.Padmanabhan,J.Mater.Sci.31(1996)4381–4385.[9]H.Wohlfarht,in:H.O.Fuchs(Ed.),Proceedings of the SecondInternational Conference on Shot Peening(ICSP-2),American Shot Peening Society,New Jersey,1984,pp.306–315.[10]E.Harrington Jr.,Industrial Quality Control21(10)(1965)494–498.[11]G.Derringer,R.Suich,J.Qual.Technol.12(4)(1980)214–219.[12]O.V ohringer,T.Hirsch,E.Macherauch,Titanium science and tech-nology,in:G.Luetjering,W.Zwicker,W.Bunk(Eds.),Fifth Inter-national Conference on Titanium,Deutsche Gesellschaft fur Metal-lkunde E.V.,vol.4,Oberusel,1984,pp.2203–2210.[13]O.V ohringer,Advances in Surface Treatment Technology,Applica-tions.[14]D.F.Neal,P.A.Blenkinsop,Titanium and Titanium Alloys,in:J.C.Williams,A.Belov(Eds.),Proceedings of Third International Conference on Titanium,vol.3,Plenum Press,New York,1982, pp.2003–2014.[15]A.L.Esquivel,R.Evans,Exp.Mech.8(1968)496.。