B Decays as a Probe of Spontaneous CP-Violation in SUSY Models
cy and medusa 翻译

cy and medusa 翻译Cy and Medusa are two characters from Greek mythology.Cy (also known as Cyclops) is a mythical creature with a single eye in the middle of its forehead. The word "Cyclops" in Greek means "round eye." In mythology, Cyclopes were giant beings with incredible strength and were known for their craftsmanship. They were said to have helped the gods build their palaces and weapons. For example, in Homer's Odyssey, the Cyclops Polyphemus is encountered by Odysseus and his men.Medusa, on the other hand, is a well-known monster in Greek mythology. She is often depicted as a creature with snakes for hair and a gaze that turns anyone who looks at her into stone. Medusa was originally a beautiful woman, but she was cursed by the goddess Athena after she was raped by Poseidon in Athena's temple. Medusa's head became a powerful weapon, capable of turning anyone who looked at it into stone. In mythology, Medusa was eventually killed by the hero Perseus.Here are a few examples of usage and translations:1. Cy and Medusa are prominent characters in Greek mythology.《赛尔与美杜莎》是希腊神话中重要的角色。
用初中英语简要介绍双缝实验

用初中英语简要介绍双缝实验The Double-Slit ExperimentThe double-slit experiment is a fundamental experiment in quantum mechanics that demonstrates the wave-particle duality of light and other quantum particles. It was first performed by the English physicist Thomas Young in 1801, and it has since become one of the most famous experiments in the history of science.The basic setup of the double-slit experiment is as follows. A source of light, such as a laser or a monochromatic light source, is directed towards a barrier that has two narrow slits cut in it. The light passing through the slits is then projected onto a screen or a detector. When the light passes through the two slits, it creates an interference pattern on the screen, with alternating bright and dark regions.This interference pattern is a clear demonstration of the wave-like nature of light. If light were simply a stream of particles, one would expect to see two separate bright spots on the screen, corresponding to the two slits. However, the interference pattern shows that the light is behaving like a wave, with the waves from the two slits interfering with each other.The double-slit experiment can also be performed with other quantum particles, such as electrons or atoms. When these particles are directed towards the double slit, they also exhibit an interference pattern, indicating that they too have a wave-like nature.The wave-particle duality of quantum particles is a fundamental concept in quantum mechanics. It means that particles can exhibit both wave-like and particle-like properties, depending on the experiment being performed. This is a departure from the classical view of the world, where objects were either waves or particles, but not both.The double-slit experiment has been used to demonstrate the wave-particle duality of various quantum particles, including electrons, neutrons, atoms, and even large molecules. In each case, the interference pattern observed on the screen is a clear indication of the wave-like nature of the particles.One of the most interesting aspects of the double-slit experiment is the role of the observer. When the experiment is set up to detect which slit the particle goes through, the interference pattern disappears, and the particles behave like classical particles. This suggests that the act of measurement or observation can affect the behavior of quantum particles.This is a concept known as the "observer effect" in quantum mechanics, and it has profound implications for our understanding of the nature of reality. It suggests that the very act of observing or measuring a quantum system can alter its behavior, and that the observer is not a passive participant in the experiment.The double-slit experiment has also been used to explore the concept of quantum entanglement, which is another fundamental concept in quantum mechanics. Quantum entanglement occurs when two or more quantum particles become "entangled" with each other, such that the state of one particle is dependent on the state of the other.In the double-slit experiment, the interference pattern can be used to demonstrate the phenomenon of quantum entanglement. For example, if two particles are entangled and then directed towards the double slit, the interference pattern observed on the screen will depend on the state of the entangled particles.Overall, the double-slit experiment is a powerful and versatile tool for exploring the fundamental nature of reality at the quantum level. It has been used to demonstrate the wave-particle duality of light and other quantum particles, the observer effect, and the phenomenon of quantum entanglement. As such, it remains one ofthe most important and influential experiments in the history of science.。
外星文明猜想(英文作文)

Certainly! Here’s an essay exploring the conjectures about extraterrestrial civilizations, delving into the scientific, philosophical, and speculative aspects of the topic. Extraterrestrial Civilizations: The Great Beyond and Our Place in the CosmosThe universe, vast and ancient, stretches its arms across 93 billion light-years of observable space, containing billions of galaxies, each with billions of stars. Within this cosmic tapestry, the question of whether we are alone has captivated human minds for centuries. This essay explores the conjectures surrounding extraterrestrial civilizations, from the scientific theories to the speculative musings that fuel our imaginations.The Drake Equation: A Mathematical Framework for SpeculationAt the heart of the search for extraterrestrial intelligence (SETI) lies the Drake equation, formulated by astronomer Frank Drake in 1961. This mathematical framework attempts to estimate the number of active, communicative civilizations in the Milky Way galaxy. Variables include the rate of star formation, the fraction of stars with planetary systems, the number of planets capable of supporting life, the fraction of those planets where life actually emerges, the fraction of those life-bearing planets that develop intelligent life, the fraction of those that develop a civilization with technology, and the length of time such civilizations release detectable signals into space. While many of these variables remain unknown, the Drake equation serves as a tool for structured speculation and highlights the immense challenge in estimating the likelihood of extraterrestrial life.The Fermi Paradox: Where Are They?The Fermi paradox, named after physicist Enrico Fermi, poses a compelling question: Given the vastness of the universe and the high probability of habitable worlds, why have we not encountered any evidence of extraterrestrial civilizations? This paradox has led to numerous hypotheses. Perhaps civilizations tend to destroy themselves before achieving interstellar communication. Or, advanced civilizations might exist but choose to avoid contact with less developed species, adhering to a cosmic form of the “prime directive” seen in science fiction. Alternatively, the distances between stars could simply be too great for practical interstellar travel or communication, making detection exceedingly difficult.The Search for TechnosignaturesIn the quest for extraterrestrial intelligence, scientists have focused on detecting technosignatures—signs of technology that might indicate the presence of a civilization elsewhere in the universe. These include radio signals, laser pulses, or the dimming of stars due to megastructures like Dyson spheres. SETI projects, such as the Allen Telescope Array and Breakthrough Listen, scan the skies for anomalous signals that could be attributed to alien technology. While no definitive technosignatures have been found to date, the search continues, driven by advances in technology and a growing understanding of the cosmos.Astrobiology: Life Beyond EarthAstrobiology, the study of the origin, evolution, distribution, and future of life in the universe, offers insights into the conditions necessary for life. Research in astrobiology has revealed that life can thrive in extreme environments on Earth, suggesting that the conditions for life might be more widespread in the universe than previously thought. The discovery of exoplanets in the habitable zones of their stars, where liquid water can exist, increases the probability of finding environments suitable for life.Continued exploration of our solar system, particularly of Mars and the icy moons of Jupiter and Saturn, holds promise for uncovering signs of past or present microbial life. The Philosophical ImplicationsThe possibility of extraterrestrial civilizations raises profound philosophical questions about humanity’s place in the universe. Encountering another intelligence would force us to reevaluate our understanding of consciousness, culture, and ethics. It could lead to a new era of global unity as humanity comes together to face the challenges and opportunities of interstellar diplomacy. Conversely, it might also highlight our vulnerabilities and prompt introspection on our stewardship of the planet and our responsibilities as members of the cosmic community.Concluding ThoughtsWhile the existence of extraterrestrial civilizations remains a conjecture, the pursuit of answers has expanded our understanding of the universe and our place within it. The search for life beyond Earth is not just a scientific endeavor; it is a philosophical journey that challenges us to consider our origins, our destiny, and our role in the vast cosmic drama unfolding around us. Whether we find ourselves alone or part of a galactic community, the quest for knowledge about the universe and our place in it is one of humanity’s most enduring and inspiring pursuits.This essay explores various aspects of the conjectures surrounding extraterrestrial civilizations, from the scientific frameworks used to estimate their likelihood to the philosophical implications of their existence. If you have specific areas of interest within this broad topic, feel free to ask for further elaboration! If you have any further questions or need additional details on specific topics related to extraterrestrial life or astrobiology, please let me know!。
Probing R-violating top quark decays at hadron colliders

a r X i v :h e p -p h /0007280v 1 25 J u l 2000AMES-HET-00-06July 2000Probing R-violating top quark decays at hadron colliders K.J.Abraham a ,Kerry Whisnant a ,Jin Min Yang b ,Bing-Lin Young a,b a Department of Physics and Astronomy,Iowa State University,Ames,Iowa 50011,USA b Institute of Theoretical Physics,Academia Sinica,Beijing 100080,China ABSTRACT We examine the possibility of observing exotic top quark decays via R-violating SUSY interactions at the Fermilab Tevatron and CERN LHC.We present cross-sections for t ¯t production followed by the subsequent decay of either t or ¯t via the R-violating interaction while the other undergoes the SM decay.With suitable kinematic cuts,we find that the exotic decays can possibly be detected over standard model backgrounds at the future runs of the Tevatron and LHC,but not at Run 1of the Tevatron dueto limited statistics.Discovery limits for R-Violating couplings in the top sector are presented.1IntroductionThe top quark,with a mass of the order of the electroweak symmetry breaking scale,is naturally considered to be related to new physics.Run1of the Fermilab Tevatron has small statistics on top quark events and thus leaves plenty of room for new physics to be discovered at the upgraded Tevatron[1]in the near future.Due to higher statistics, the t¯t events at the upgraded Tevatron are expected to provide sensitive probes for new physics[2].The most popular model for new physics is the Minimal Supersymmetric Model(MSSM)[3].In this model,R-parity[4],defined by R=(−1)2S+3B+L with spin S,baryon-number B and lepton-number L,is often imposed on the Lagrangian to maintain the separate conservation of B and L.As a consequence the sparticle number is conserved.Since instanton effects induce miniscule violations of baryon and lepton number[5],R-parity conservation is not dictated by any known fundamental principle such as gauge invariance or renormalizability.If R-parity is strictly conserved,it is conceivable that the conservation comes from some hitherto unidentified fundamental principle.Hence R-parity violation should be vigorously searched for.The most general superpotential of the MSSM,consistent with the SU(3)×SU(2)×U(1)symmetry,supersymmetry,and renomalizability also contains R-violating interac-tions which are given byW R=12λ′′ijkǫαβγU c iαD c jβD c kγ+µi L i H2,(1) where L i(Q i)and E i(U i,D i)are the left-handed lepton(quark)doublet and right-handed lepton(quark)singlet chiral superfields.The indicies i,j,k are generation indices,α,βandγare the color indices,c denotes charge conjugation,andǫαβγis the total antisym-metric tensor in three-dimension.H1,2are the Higgs-doublets chiral superfields.The coefficientsλandλ′are the coupling strengths of the L-violating interactions andλ′′those of the B-violating interactions.The lower bound of the proton lifetime imposes very strong conditions on the simultaneous presence of both L-violating and B-violating interactions[6]and hence the strength of the couplings.However,the existence of either L-violating or B-violating couplings,but not both at the same time,does not induce nucleon decays and therefore the R-parity violating couplings are less constrained.This separate L and B violation is usually assumed in phenomenological analyses.The study of the phenomenology of R-violating supersymmetry was started many years ago[7].Some constraints on the R-parity violating couplings have been obtained from various analyses,such as perturbative unitarity[8],n−¯n oscillation[9],νe-Majoranamass[10],neutrino-less doubleβdecay[11],charged current universality[12],e−µ−τuniversality[12],νµ−e scattering[12],atomic parity violation[12],νµdeep-inelastic scat-tering[12],µ−e conversion[13],K-decay[16],τ-decay[15],D-decay[15],B-decay[16] and Z-decay at LEP I[17].As reviewed in Ref.[18],although many such couplings have been severely constrained,the bounds on the top quark couplings are generally quite weak.This is the motivation for the phenomenological study of R-violation in processes involving the top quark.The production mechanisms of top pairs and single top in R-violating SUSY at the upgraded Tevatron have been examined in[19]and[20],respectively.In addition,the R-violating couplings can induce exotic decays for top quark at an observable level. For example,the top quark FCNC decays induced by R-violating couplings[21]can be significantly larger than those in the MSSM with R-parity conservation[22].If we allow the co-existence of twoλ′couplings,we have the new decay modes,such as t→ℓ˜d→ℓ+ℓ−u[23].The bilinear termµi L i H2can also induce some new decays for the top quark,as studied in[24].In this work,we focus on the explicit trilinear couplings and assume only one trilinear coupling exists at one time.Then the possible exotic top decay modes aret→˜¯d i¯d j,˜¯d j¯d i→¯d i¯d j˜χ01(2) induced by the B-violatingλ′′3ij,andt→e+i˜d j˜e i d j→e+i d j˜χ01(3) induced by the L-violatingλ′i3j.Here the subscripts i,j are family indices and˜χ01is the lightest neutralino which,in our analysis,is assumed to be the lightest super particle (LSP)as favored in the MSSM where the SUSY breaking is propagated to the matter sector by gravity1.The sfermions involved in these decays can be on-shell or virtual, depending on the masses of the particles involved.Among the exotic decays in(2)and(3),the relatively easy-to-detect modes are those induced byλ′′33j(j=1,2)2andλ′i33(i=1,2,3)because theirfinal states contain a b-quark which can be tagged.One of the L-violating channels,i.e.,t→˜τb(orτ˜b)induced byλ′333has been studied in[25].So in our analysis we focus on the cases ofλ′133and λ′233for L-violating couplings,andλ′′331andλ′′332for B-violating couplings.Since the decay induced byλ′133has the similarfinal states to that induced byλ′233,we take thepresence ofλ′233as an example.For the same reason,we take the presence ofλ′′331as an example in B-violating case.The Feynman diagrams for these two decays induced by λ′′331andλ′233are shown in Figs.1and2,respectively.In our analysis we consider t¯t events where one(t or¯t)decays via R-violating coupling while the other decays by the SM interaction.The SM decay will serve as the tag of the ¯t t event.Furthermore,the penalty of the suppressed R-violation coupling is paid only once.Top spin correlations are taken into account in our calculation.Note that the LSP(˜χ01)is no longer stable when R-parity is violated.In case just one R-violating top quark coupling does not vanish,the lifetime of the LSP will be very long,depending the coupling and the masses of squarks involved in the LSP decay chain(cf.the last paper of[18]).Thus it is generally assumes that the LSP decays outside the detector[26].We will make this assumption in our analysis.This paper is orgnized as follows.In Sec.2,we investigate the potential of observing the B-violating top quark decay at the Tevatron and LHC,and present numerical results.In Sec.3we present similar results for L-violating decay.Finally in Sec.4we present a summary and discussion.2Searching for B-violating decay2.1Signal and backgroundTo probe the decay t→¯b¯d˜χ01in Fig.1,we consider thefinal states given by t¯t production where one(say t)decays via the couplingλ′′331while the other(say¯t has the SM decays to serve as the tag of the¯t t event.Due to the large QCD background at hadron colliders,we do not search for the all-jets channel despite of its higher rate.Instead,we search for the signal given by t¯t events followed by t→¯b¯d˜χ01and¯t→W−¯b→ℓ¯ν¯b(ℓ=e,µ).Then the signature is a lepton,three jets containing two b-jets or two¯b-jets,and missing energy (ℓ+3j/2b+E T).We require that two b-jets are tagged in the signal.The efficiency for double b-tagging is assumed to be42%[1].Note that the present events have the unique signal of the two same sign b-quarks. In our analysis,to be conservative,we assume that the tagging can not distinguish a b-quark jet from¯b-quark jet.Then the SM backgrounds are mainly from(1)t¯t→W−W+b¯b followed by W−→ℓ¯ν(ℓ=e,µ)and W+→τ+νwith theτdecaying into a jet plus a neutrino;(2)t¯t→W−W+b¯b followed by W−→ℓν(ℓ=e,µ)and W+→q¯q′.This processcontains an extra quark jet and can only mimic our signal if the quark misses detection by going into the beam pipe.We assume this can only happen when the light quark jet has the pseudo-rapidity greater than about3or the transverse momentum less than about10GeV.(3)W b¯bj which includes single top quark production via the quark-gluon processqg→q′t¯b as well as non-top processes[27].2.2Numerical calculation and resultsWe calculated the signal and background cross sections with the CTEQ5L structure√functions[28].We assume M t=175GeV and takes=14TeV for the LHC.As shown in Fig.1,there are two contributing graphs.Since among the down-type squarks the sbottom is most likely to be lighter than other squarks(we will elaborate on this later),we assume thefirst graph in Fig.1gives the dominant contribution.(If the ˜d is as light as the sbottom,the second diagram in Fig.1has to be taken into account. Then our results for the signal rate should be quadrupled.To be conservative,we do not consider this case.)For the total width of the sbottom involved in our calculation,we note that since only a light sbottom is meaningful to our analysis(as will be shown in our results), its dominant(or maybe the only)decay mode is˜b→b˜χ01.The charged current decay mode˜b→t˜χ+1is kinematically forbidden for a light sbottom in our analysis.We do not consider the strong decay mode˜b→b˜g since the gluino˜g is likely to be heavy[29]. The signal cross section is proportional to|λ′′331|2.We will present the signal results normalized to|λ′′331|2.The signal cross section is very sensitive to the sbottom mass. We will vary it to see how heavy it can be for the signal to be observable.Other SUSY parameters involved are the lightest neutralino mass and its coupling to sbottom,which are determined by the parameters M,M′,µand tanβ.M is the SU(2)gaugino mass and M′is the hypercharge U(1)gaugino mass.µis the Higgs mixing term(µH1H2) in the superpotential.tanβ=v2/v1is the ratio of the vacuum expectation values of the two Higgs doublets.We work in the framework of the general MSSM.But we assume the grand unification of the gaugino masses,which gives the relation M′= 5choose a representative set of valuesM=100GeV,µ=−200GeV,tanβ=1.(4)They yield the lightest chargino and neutralino masses as m˜χ+1=120GeV,m˜χ01=55GeV.Thus this set of values are allowed by the current experimental bounds on the chargino and neutralino masses,which are about90GeV and45GeV,respectively[30].We simulate detector effects by assuming Gaussian smearing of the energy of the chargedfinal state particles,given by:∆E/E=30%/√E⊕5%,for hadrons,(6)where⊕indicates that the energy dependent and independent terms are added in quadrature and E is in GeV.The basic selection cuts are chosen aspℓT,p jet T,p missT≥20GeV,(7)ηjet,ηℓ≤2.5,(8)∆R jj,∆R jℓ≥0.5.(9) Here p T denotes transverse momentum,ηis the pseudo-rapidity,and∆R is the separa-tion in the azimuthal angle-pseudo rapidity plane(∆R=(| PℓT|+| P miss T|)2−( PℓT+ P miss T)2.(10)As is well-known,if the two components,i.e.,ℓand p missTin our case,are from the decay of a parent particle,the transverse mass is bound by the mass of the parent particle.Sofor W b¯bj background events m T(ℓ,p missT)is always less than M W and peaks just below M W.However,kinematic smearings can push the bound and the peak above M W.In order to substantially suppress the large backgrounds(2)and(3)we apply the following cutm T(ℓ,p missT)>120GeV.(11)We found that the above strong m T(ℓ,p miss)cut suppresses the background process(2)Tand(3)by roughly three orders of magnitude for the smearing in Eqs.(5)and(6),sothat they are much smaller than the other backgrounds we are considering.But since background process(1)contains three neutrinos from different parent particles,it is not supressed by the m T(ℓ,p miss)cut to a negligible level.There is some model dependenceTinvolved the treatment ofτhadronization.To avoid having to consider each of the many hadronic decay modes separately,we assume the invariant mass of the outgoing hadrons to be distributed uniformly from mπto mτ.Furthermore,we assume a uniform angular distribution in the phase allowed by the invariant mass of the outgoing jet.This assumption is probably reasonable in light of the fact that the parentτis heavily boosted in the lab frame.With the above selection cuts,the signal and background cross sections are given in Table1.We see that the signal-to-background ratio can be quite large for light sbottom mass(∼<160GeV),in which the intermediate sbottom can be materialized as a real particle.When the sbottom becomes heavier than the top quark and thus can only appear as a virtual state,the cross section is severely suppressed by the small branching ratio of the decay.From the results for Tevatron(1.8TeV)in Table1we conclude that the luminosity Run1(0.1fb−1)is too low to detect such decays.However,due to the much largerstatistics of Run2(2fb−1)and Run3(30fb−1),it is possible to observe such decays in√these coming runs of the ing the discovery criteria S≥5changing a slepton.As in Sec.2,we assume sbottom can be light and thus concentrate on thefirst graph.In the opposite case that the slepton is light and sbottom is heavy, our following results still hold with the replacement of sbottom mass by slepton mass. If both sbottom and slepton are light and approximately degenerate(which is quite un-likely in the supergravity scenario of supersymmetry breaking,as will be elaborated on later),then our results for the signal rate should be quadrupled.Our examination for this decay is similar to the B-violating decay in the preceding section.We search for the signal given by t¯t events where one(say t)decays via L-violating coupling,t→µ+b˜χ01,while the other(¯t)has the SM decays,¯t→W−¯b.Then there are two possible observing channels for such an event:dilepton+2-jets and single lepton+4-jets,all being associated with missing energies.The dilepton channel has the lower rate and it is difficult tofind a mechanism to enhance the S/B rate so as tofind the ”smoking gun”for the signal.So we search for the single lepton+4-jets channel which has a higher rate.As is shown below,we canfind effective selection cuts to enhance the S/B ratio for this signal.Among the four jets in our signal there are two b-jets(one is b,the other is¯b).We require that at least one b-jet passes b-tagging.The tagging efficiency is53%at Run1 and expected to reach85%at Run2and Run3[1].For the LHC we assume the tagging efficiency to be the same as the Tevatron Run2.So the signature isℓ+4j/b+E T where4j/b represents a4-jets event with at least one of the jets passing the b-tagging criterion.This is the same as one of the typical signatures for t¯t event in the SM,except for the different source of missing energy.To suppress the QCD background,we apply the basic selection cuts in Eqs.(7-9).Under the basic selection cuts the QCD background is reduced to about1/12of the SM t¯t events[1].However,under the basic selection cuts the number of SM t¯t events far surpasses the number of signal events.In order to extract the signal events,we turn to the transverse mass defined in Eq.(10).For the SM t¯t events and W+jets background events the missing energy comes from the neutrino of the W decay,while for the signal events the missing energy comes from the neutralino in the decay t→µ+˜b→µ+b˜χ01. Thus the transverse mass distributions of the SM background and the signal events are different,as shown in Fig.5.In order to enhance the S/B ratio,we apply the following cut,taking into account of the smearing effect,m T(ℓ,p miss)∈50∼100GeV.(12)TOther details in the numerical calculation,such as the smearing of the energy ofthefinal state particles and the choice of SUSY parameters,are the same as in Sec.2. In Table2we present the signal cross section for sbottom mass of150GeV,with the comparison to the SM t¯t background.One sees that the transverse mass cut can enhance the S/B ratio significantly.With the increase of sbottom mass,the signal cross section drops rapidly,as shown in Table3.From Tables2and3one sees that Run1(0.1fb−1)of the Tevatron collider is unable to detect such decays for a sbottom heavier than150GeV andλ′233<1.The possibility of observing such a decay is enhanced at Run2(2fb−1),Run3(30fb−1)and the LHC.√Under the discovery criteria S≥5A few remarks are due regarding our results:(1)The results are sensitive to the sbottom mass;the signal is observable only for alight sbottom.The possibility of a light sbottom is usually motivated as follows: Firstly,the neutral kaon system gives a strong constraint[31]on the masses of thefirst and second generation squarks.The third generation sfermions are much less constrained so far.Secondly,in the supergravity scenario of supersymmetry breaking,mass splitting of the third-generation and the other sfermions results from the renormalization group evolution of the masses between the unification scale and the weak scale,even if the sfermions have equal masses at the unification scale.This splitting is due to the effect of the large Yukawa coupling of the top.The bottom and tau sectors are also affected.Thirdly,there are arguments[32] thatfirst and second generation sfermions can be as heavy as10TeV without conflicting the naturalness problem,while the third generation sfermions have to be rather light.(2)As pointed out in Sec.(1),the two decay processes we considered resemble thefavorable cases in which a b-jet is produced in the decay products.While we can apply our results directly to the cases ofλ′′332andλ′133,we noticed that similar decays induced by other couplings likeλ′′312andλ′232give poor signals since there is no b-quark in their corresponding top decays.(3)We noted that apart from the relevant R-violating couplings and the sbottom mass,our results are also dependent on the mass and coupling of the lightest neutralino.In our calculation we only present some illustrative results byfixing a set of SUSY parameters rather than scanning the entire allowed SUSY parameter space.In some unfavorable cases,such as when the mass of the lightest neutralino(LSP)is close to the sbottom mass so that the b-quark from the sbottom decay(˜b→b˜χ01) is too soft to pass the selection cuts,these exotic decays would be unobservable even at the LHC.(4)As pointed out in Sec.2,the B-violating decay gives the unique signal of samesign b-quarks while the main SM backgrounds give the unlike sign b-quarks.To be conservative,we assumed in our analysis that the b tagging is not of sufficient sensitivity to distinguish between a b-jet and a¯b-jet.If b charge identification can be achieved in future detectors,more stringent discovery limits than those we have presented will be possible.Additional improvements will be possible if hadronicjets fromτdecays can be clearly identified as such,thus reducing the background fromτhadronization.AcknowledgmentsWe thank E.Boos and L.Dudko for the discussion of W b¯bj background.BLY acknowl-edges the hospitality extended to him by Professor Zhongyuan Zhu and colleagues at the Institute of Theoretical Physics,Academia Sinica,where part of the work was per-formed.This work is supported in part by a grant of Chinese Academy of Science for Outstanding Young Scholars and also by a DOE grant No.DE-FG02-92ER40730.References[1]Report of the tev(1986);H.Dreiner,G.G.Ross,Nucl.Phys.B365,597(1991);H.Dreiner,R.J.N.Phillips,Nucl.Phys.B367,591(1991).[8]B.Brahmachari and P.Roy,Phys.Rev.D50,39(1994);L.Goity and M.Sher,Phys.Lett.B346,69(1995).[9]F.Zwirner,Phys.Lett.B132,103(1983).[10]S.Dimopoulos and L.J.Hall,Phys.Lett.B207,210(1987);R.M.Godbole,P.Roy and X.Tata,Nucl.Phys.B401,67(1993).[11]R.N.Mohapatra,Phys.Rev.D34,3457(1986);M.Hirsch,H.V.Klapdor-Kleingrothaus,S.G.Kovalenko,Phys.Rev.Lett.75,17(1995);K.S.Babu and R.N.Mohapatra,Phys.Rev.Lett.75,2276(1995).[12]V.Barger,G.F.Giudice and T.Han,Phys.Rev.D40,2978(1989).[13]K.Huitu,J.Maalampi,M.Raidal,A.Santamaria,Phys.Lett.B430,355(1998).[14]K.Agashe and M.Graesser,Phys.Rev.D54,4445(1996);D.Choudhury and P.Roy,hep-ph/9603363.[15]G.Bhattacharyya and D.Choudhury,Mod.Phys.Lett.A10,1699(1995).[16]D.E.Kaplan,hep-ph/9703347;J.Jang,J.K.Kim and J.S.Lee,hep-ph/9701283;hep-ph/9704213;Phys.Rev.D55,7296(1997);T.-F.Feng,hep-ph/9806505;C.H.Chang,T.-F.Feng,hep-ph/9908295.[17]J.M.Yang,hep-ph/9905486;G.Bhattacharyya,J.Ellis and K.Sridhar,Mod.Phys.Lett.A10,1583(1995);G.Bhattacharyya,D.Choudhury and K.Sridhar, Phys.Lett.B355,193(1995).[18]G.Bhattacharyya,hep-ph/9709395;H.Dreiner,hep-ph/9707435;S.Raychaudhuri,hep-ph/9905576;R.Barbier et al,hep-ph/9810232.B.Allanach et al,edited byH.Dreiner,hep-ph/9906224.[19]A.Datta,J.M.Yang,B.-L.Young and X.Zhang,Phys.Rev.D56,3107(1997);R.J.Oakes,K.Whisnant,J.M.Yang,B.-L.Young and X.Zhang,Phys.Rev.D57, 534(1998);P.Chiappetta,A.Deandrea,E.Nagy,S.Negroni,G.Polesello,J.M.Virey,Phys.Rev.D61,115008(2000).[20]K.-I.Hikasa,J.M.Yang,B.-L.Young,Phys.Rev.D60,114041(1999);D.K.Ghosh,S.Raychaudhuri and K.Sridhar,Phys.Lett.B396,177(1997).[21]C.S.Li,R.J.Oakes and J.M.Yang,Phys.Rev.D49,293(1994);G.Couture,M.Frank and H.Konig,Phys.Rev.D56,4213(1997);G.M.de Divitiis,R.Petronzio, L.Silvestrini,Nucl.Phys.B504,45(1997);J.L.Lopez,D.V.Nanopoulos,R.Ran-garajan,Phys.Rev.D56,3100(1997).[22]J.M.Yang,B.-L.Young and X.Zhang,Phys.Rev.D58,055001(1998);S.Bar-Shalom,G.Eilam,A.Soni,Phys.Rev.D60,035007(1999).[23]D.Atwood,talk at“Thinkshop On Top Quark Physics Of Run II”,FNAL,Oct.16-18,1998(web address /thinkshop/).[24]F.Campos,et al.,hep-ph/9903245,to appear in the proceedings of the workshop“Physics at Run II:SUSY/Higgs”,Fermilab,Nov.,1998.[25]M.B.Magro and T.Han,talk at“Thinkshop On Top Quark Physics Of Run II”,FNAL,Oct.16-18,1998(web address /thinkshop/).[26]See,for example,E.L.Berger,B.W.Harris,Z.Sullivan,Phys.Rev.Lett.83,4472(1999).[27]E.Boos,L.Dudko,T.Ohl,Eur.Phys.J.C11,473(1999).[28]i,et al.,hep-ph/9903282.[29]CDF collaboration,Phys.Rev.D56,R1357(1997);D0collaboration,EPS-HEPConf.,Jerusalem(1997)Ref.102.[30]LEP2SUSY Working Group,http://www.cern.ch/lepsusy.[31]J.Ellis and D.V.Nanopoulos,Phys.Lett.B110,44(1982); F.Gabbiani,E.Gabrielli,A.Masiero,and L.Silvestrini,Nucl.Phys.B477,321(1996);J.A.Bagger,K.T.Matchev,and R.-J.Zhang,Phys.Lett.B412,77(1997).[32]M.Dine,A.Kagan,and S.Samuel,Phys.Lett.B243,250(1990);S.Dimopoulosand G.F.Giudice,Phys.Lett.B357,573(1995);A.Pomarol and D.Tommasini, Nucl.Phys.B466,3(1996);A.Cohen,D.B.Kaplan,and A.E.Nelson,Phys.Lett.B388,599(1996).N.Arkani-Hamed and H.Murayama,Phys.Rev.D56,R6733 (1997).Table1:Signalℓ+3j/2b+E T and background cross sections in units of fb.The basic cuts are p all T≥20GeV,|ηall|≤2.5and∆R≥0.5,and the transverse mass cut is m T≥120GeV.The signal results were calculated by assuming M=100GeV,µ=−200GeV and tanβ=1. The double b-jet tagging with42%efficiency is assumed.The charge conjugate channels have been included.Signal/(λ′′331)211 5.8 2.040.270.010.0050.003(1.8Tev)Signal/(λ′′331)2168.4 3.00.40.020.0070.004 (2Tev)Signal/(λ′′331)2162488537158 1.70.40.3 (14Tev)Table2:Signalℓ+4j/2b+E T and the SM t¯t background cross sections for sbottom mass of 150GeV.The basic cuts are p all T≥20GeV,|ηall|≤2.5and∆R≥0.5,and the transversemass cut is m T(ℓ,p missT)∈50∼100GeV.The signal results were calculated by assuming M=100GeV,µ=−200GeV and tanβ=1.Tagging at least one b-jet is assumed for53% efficiency for the Tevatron(1.8TeV),85%efficiency for the upgraded Tevatron(2TeV)and LHC.The charge conjugate channels have been included.basic cutsm T(ℓ,p missT )cutSignal/(λ′233)2(fb)43 Background(fb)86 Signal/(λ′233)2(fb)96 Background(fb)193 Signal/(λ′233)2(pb)8.2 Background(pb)16Table3:Same as Table2,but for the signal cross section versus sbottom mass under the basic plus transverse mass cut.Tevatron(1.8Tev):150155160165170180190200 Signal/(λ′331)2(fb)Tevatron(2TeV):150155160165170180190200 Signal/(λ′233)2(fb)LHC(14TeV):150155160165170180190200 Signal/(λ′′233)2(pb)Figure1:The Feynman diagram for the B-violating decay induced byλ′′331.Figure2:The Feynman diagram for the L-violating decay induced byλ′233.Figure3:The discovery limits ofλ′′33j versus sbottom mass at Run2(2fb−1),Run3 (30fb−1)and LHC(10fb−1).The region above each curve is the corresponding regionof discovery.Figure4:The exclusion limits ofλ′′33j versus sbottom mass at Run2(2fb−1),Run3 (30fb−1)and LHC(10fb−1).The region above each curve is the corresponding regionof exclusion.Figure5:The transverse mass,m T(ℓ,p missT ),distribution ofℓ+4j/b+E T at theTevatron collider.The solid curve is for the signal event.The dotted curve is for the SM t¯t background.Figure6:The discovery limits ofλ′233versus sbottom mass at Run2(2fb−1),Run3 (30fb−1)and LHC(10fb−1).The region above each curve is the corresponding regionof discovery.Figure7:The exclusion(95%C.L.)limits ofλ′233versus sbottom mass at Run2(2fb−1), Run3(30fb−1)and LHC(10fb−1).The region above each curve is the correspondingregion of exclusion.。
老师看不出是抄的我的奇思妙想英语作文

老师看不出是抄的我的奇思妙想英语作文全文共3篇示例,供读者参考篇1My Wildest Dreams and FantasiesYou know that feeling when your mind starts to wander and suddenly the most peculiar thoughts take over? It's like being transported to another dimension where reality is bent and anything is possible. Well, let me take you on a journey through the untamed wilderness of my imagination. Buckle up, because this ride is about to get weird!Imagine a world where dogs could talk. Not just bark or whine, but engage in full-blown conversations. I'm picturing myself strolling through the park, casually chatting with a pack of Golden Retrievers about the latest bone-chilling thriller they've been digging into (pun very much intended). We'd debate the finer points of storytelling, critique the character development, and maybe even exchange a few doggy puns for good measure. Who knows, I might even get some writing tips from thesefour-legged literary critics!Now, let's take a sharp left turn into the realm of the utterly absurd. What if clouds were made of cotton candy? Imagine gazing up at the sky, watching those fluffy, sugary formations drifting lazily overhead. I can almost taste the sweetness on my tongue as I reach up to pluck a handful of cloud-candy, savoring the delightful flavor bursting in my mouth. Of course, this could lead to some sticky situations – quite literally. Rainclouds would shower us with sugary syrup, and thunderstorms would be an absolute mess. But hey, at least we'd never run out of dessert!Speaking of food, have you ever wondered what it would be like if vegetables could scream? Imagine the horror of biting into a juicy tomato, only to be met with a blood-curdling shriek emanating from its fleshy interior. Chopping an onion would become a form of cruel and unusual punishment, unleashing a torrent of anguished wails. Cooking would be a traumatic experience, filled with the agonized cries of defenseless veggies begging for mercy. On second thought, maybe we should stick to a strict candy diet in this fantastical world.Now, let's drift into the realm of the truly outlandish. What if the moon was made of cheese? Not just any cheese, mind you, but the gooiest, most mouth-watering cheddar you can imagine. Imagine gazing up at that golden, celestial disc, its craters filledwith pools of melted deliciousness. Astronauts would be replaced by cheese connoisseurs, embarking on expeditions to mine the lunar surface for the finest, most aged varieties. The only downside? Lunar eclipses would be a real letdown, blotting out that tantalizing sight with the dull hunk of rock we call Earth.But wait, there's more! How about a world where gravity was reversed? Instead of being pulled down, we'd find ourselves constantly floating upwards, desperately clinging to anything anchored to the ground. Imagine the chaos of trying to have a casual conversation, both parties drifting lazily towards the ceiling as they exchange pleasantries. Eating would be a messy affair, with food and utensils constantly escaping our grasp and disappearing into the stratosphere. And let's not even talk about the challenges of using the restroom in such an environment. On second thought, maybe we should just stick to the ground for that particular activity.Of course, no journey through the bizarre would be complete without exploring the idea of animals walking on their hind legs. Picture yourself strolling down the street, only to encounter a group of upright dogs engaged in a lively discussion about the latest trends in chew toys. Or perhaps you'd stumble upon a gathering of bipedal cats, sipping daintily from tinyteacups as they gossip about the neighborhood's most eligible toms. It would be a surreal sight, to say the least, but also strangely endearing in its own peculiar way.As our adventure through the whimsical reaches of my mind comes to a close, I can't help but feel a sense of wonder and excitement. The ability to imagine the impossible, to dream up scenarios that defy logic and reason, is what makes the human experience so rich and fascinating. So, the next time your thoughts start to wander down an unconventional path, don't shy away from the absurdity. Embrace the weird, the wacky, and the downright bizarre. Who knows? You might just stumble upon your own personal wonderland, filled with talking dogs, cotton candy clouds, and a moon made of cheese.篇2My Wildest DreamsAs I lay in bed last night, staring at the glow-in-the-dark stars stuck to my ceiling, my mind started to wander. I found myself pondering the vast mysteries of the universe and imagining what incredibilities could exist out in the great cosmic unknown. The more I thought about it, the more my imagination ran wild with fanciful possibilities.What if there was another Earth-like planet out there with intelligent life forms vastly different from humans? Maybe there's a planet made entirely of candy with lollipop trees and cookie crumble mountains. Or a world with floating cities held aloft by anti-gravity technology. Anything seems possible when you really open your mind to the mysteries of existence.I started imagining myself on a journey through the cosmos, exploring strange new worlds. On the candy planet, I'd be able to bite into a chocolate mansion or drink from a river of root beer without any consequences. The floating cities would be glorious metropolises suspended in perpetual motion among the clouds and stars.Maybe there's even a planet made up of pure energy where life takes the form of concentrated beams of light and heat. How wild would it be to see cities built of plasma and lightning? I could soar effortlessly by bending radiation around me like a surfboard on a wave.As my mind continued racing with these fanciful ideas, I found myself hoping that somewhere out there, such extraordinary places truly exist. If nothing else, it's fun to imagine the possibilities of what could be.Of course, I'm not naive enough to think there's a real candy planet or cities of pure energy. But who's to say there aren't other planets with forms of life we can scarcely comprehend from our limited human perspective? With how little we know about the larger cosmos, anything seems possible if you allow your imagination to run free.I started pondering how life could evolve in ways entirely different from what we understand. On Earth, carbon-based life emerged from the primordial soup, slowly developing into complex multi-cellular organisms over billions of years. But what if a planet existed where life arose from pure energy or something entirely different and unexpected?There could be civilizations made up of sentient machines that have evolved their artificial intelligence to become a new form of technological life. Or what about a world where life emerged from complex neutron flows, forming itself into something akin to what we'd perceive as neutron stars that can somehow think and reason? The possibilities are endless when you shed your anthropocentric biases.My mind then drifted to what kind of unfathomable technologies could exist across the vastness of space and time. We humans have built some incredible devices like computers,rockets, and particle colliders. But those surely pale in comparison to what could be out there across the galaxies.I imagined a civilization that had mastered teleportation and could effortlessly travel between star systems in the blink of an eye. Or a race of beings that had unlocked the secrets of wormhole physics to create shortcuts across the cosmos. Maybe there are advanced species that long ago transcended physical forms altogether to become purely energetic beings capable of riding the very fabric of space-time itself.As a sci-fi fan, I'm quite familiar with concepts like hyperdrives, warp drives, and other fanciful means offaster-than-light travel that skirt around the cosmic speed limit of light speed. But who's to say more intelligent and ancient alien species haven't already cracked the code to achieve something like that or far crazier things we can't yet conceive?I then found myself wondering just how old some alien civilizations could truly be across this mind-bogglingly vast universe of ours. Our own human history only stretches back a few thousand years from an evolutionary standpoint. But there could be races of xenomorphs out there that are billion, if not trillions of years more ancient than we are.What unfathomable wisdom and technologies could they have amassed over such cosmically vast timescales? There's a chance some could be old enough to have originated during the earliest epochs after the Big Bang itself when the universe was an entirely different place. That's enough to make your head spin if you think about it too much.Of course, these are all wild thoughts and fanciful ideas with no grounding in scientific facts or evidence. I'm just an awestruck teenager allowing my imagination to run rampant across the celestial expanses of my mind's eye. But that's precisely what drives the wonderment of science and human curiosity.While the concepts I'm dreaming up are firmly rooted in the realms of science fiction and fantasy for now, the truth is we simply don't know what actual marvels could exist across this mind-bendingly massive universe of ours. It was once thought the sun revolved around the Earth and that our planet was the center of all creation. Just a few centuries ago, atoms and electromagnetic waves were still unknown to humankind.As our scientific understanding of the cosmos continues advancing by leaps and bounds, who's to say what shocking revelations tomorrow may bring? What we consider to be the wildest fiction today could turn out to be tame reality in thedecades and centuries to come. Keeping an open mind to the seemingly impossible is what continually expands the frontiers of knowledge and discovery.While my restless mind conjured up elaborate alien worlds and unfathomable cosmic technologies in my latest reverie, I realize how little we truly understand about this vast universe surrounding us. Our knowledge is but a drop in the ocean compared to the mysteries still left to be unraveled. But that ultimate uncertainty and sense of wonderment is what keeps driving humanity to explore, question, and push the boundaries of our perception.As my eyelids grew heavy from an all-nighter of vivid cosmic daydreaming, the last whimsical thought I remember before succumbing to sleep's embrace was: What if there's another universe out there entirely parallel to our own, filled with duplicates of every person and civilization? A vast multiverse of infinite realities coexisting across a baffling higher-dimensional expanse?Perhaps in another universe, a different version of me is writing down his own celestial reveries and wildest dreams. Or maybe the latest realms of my subconscious imagination have revealed glimpses of something startling yet to be discoveredabout this endlessly fascinating cosmos of ours. Either way, I can't wait to find out more and to keep exploring the depths of reality and fantasy alike.篇3The Cosmic DreamweaverI can still vividly recall the night everything changed – the night I met the Cosmic Dreamweaver. It was a sultry summer evening, the kind where the thick air seems to wrap around you like a stifling blanket. I had retreated to the solitude of my backyard, seeking refuge from the oppressive heat trapped inside the house. As I flopped onto the weathered wooden bench, I gazed up at the inky black canvas of the night sky, mesmerized by the twinkling diamonds strewn across its expanse.Suddenly, something caught my eye – a shooting star streaked across the heavens, burning brighter than any I'd ever witnessed before. But this was no ordinary meteor. As I watched, transfixed, the blazing streak shifted course, looping and pirouetting through the stars in mesmerizing patterns. My eyes must have been playing tricks on me, I reasoned, rubbing themfuriously. When I opened them again, the fiery trail had ceased its celestial dance and was hurtling directly towards me!I threw my arms up in a feeble attempt at self-defense, bracing for catastrophic impact. But instead of a searing demise, I felt an odd tingling spreading from the top of my head down to the soles of my feet. The tingling intensified until my entire being seemed to dissolve into a blinding kaleidoscope of color and sound. I slipped into a state of transfixed delirium, my consciousness unraveling and reweaving itself into strange new patterns.When my senses sluggishly rejoined, I found myself no longer in the confines of my backyard, but adrift in a realm of breathtaking alien splendor. Colossal spirals of multihued energy undulated all around me, emanating waves of pure harmonic resonance that reverberated through my very soul. Gargantuan celestial structures, their surfaces sculpted into fractal cities of hypnotic complexity, materialized and dematerialized in the cosmic expanse. I felt utterly minuscule, a solitary speck of near-nothingness amid the grandeur of this ineffable wonderland.As I spun about, gawking in open-mouthed awe, something drifted into my field of perception – an entity that defiedcomprehension, yet filled me with a strange sense of tranquility. It was an ever-shifting kaleidoscope of light and form, its visage cycling through a mesmerizing array of avatars: one moment an ascended astral being, brimming with arcane power; the next, a humble woodlands creature; a living fractal; a swirling nebula birth new worlds..."Do not be afraid, Earthchild," a warm voice reverberated through my psyche. "I am the Cosmic Dreamweaver. I have summoned you here to share with you the ultimate gift.""G-gift?" I stammered, utterly confounded. "What gift? Where... where is 'here?'"The entity's avatar shimmered and contorted, resolving into a wizened humanoid form that nonetheless thrummed with latent cosmic power."This place exists beyond the boundaries of your physical universe, in a realm of pure potentiality woven into the fabric of existence itself. And my gift to you is the ability to shape and reshape reality to your wildest imaginings."With an outstretched hand, the Cosmic Dreamweaver released an incandescent torrent of pure creative energy that engulfed my being. I felt my mind expanding immeasurably,blazing new neural pathways flaring open to accept the incomprehensible secrets of this strange place. Images, insights, and alien epiphanies flooded my consciousness in a deluge of pure inspiration."You now possess the power to reach into the dreamscapes of potential and pluck forth entire worlds from your thoughts," intoned the Dreamweaver. "Shape them through force of will and concentration alone into any form you can envisage. Let your imagination be your guide, and the only boundaries will be those you conceive for yourself."The being's avatar contracted into a shimmering sphere that hovered before me, dreamlike whispers echoing fromall sides: "Embrace your innermost fancies and yearnings... All is possible in the realm of dreamweavers..."With those parting words, the strange sphere imploded in a silent blossoming of spectral light. When the brilliance cleared, I found myself once again beneath the familiar night sky, on the worn bench where I'd been stargazing. Had it all been an fever dream hallucination brought on by the heat? I started to convince myself of that logical conclusion when a tinyalpha-numericticker tape began unfurlingitself from my mind'seye. It crystallized into a coherent stream of code – new neural programming granting me impossible abilities.I let the alien algorithms and fractal ciphers wash over me, integrating the incredible power into the core of my being. Taking a deep, centering breath, I focused my newly opened mindand willed my consciousness to expand outward, perceiving and interacting with the Potentiality Matrix underlying all of existence.Suddenly, the backyard scene distorted and fragmented into coruscating wings of light and shadow. A kaleidoscopic vortex spiraled into being, drawing me inward onto a vast formless expanse awash in the primal waters of creation. Here, in the nexus of conceptual potentials, my abilities as a Dreamweaver blossomed into full actualization.I imagined a twisting spire of glimmering crystal, and it effloresced before me. I envisioned floating isles crafted from woven condensed sunlight, and they shimmered into ethereal reality. Great migrating beasts with translucent bodies and metallic carapaces shimmered into existence, buoyed by levitating electrocycles that supercharged the prism-clouds that served as their propulsion...Over what seemed an eternity compressed into a singularity of subjective time, I unleashed my unbridled musings into evermore baroque expressions of pure, distilled dream-fancy. Cities crafted from living infiniglass, their axiro-latticed ziggurats straddling interdimensional continua. Vast sparkling rivers of thought that carried philosophies and mindstreams between shimmershrine arcologies. Fractal-generated megafauna forming symbiological relationships with hyper-sentient botanical canopies and roiling biomechondrial seas...At long last, my manic creative fervor found itself sated...at least for the moment. With an oblique sense of closure, I initiated a return vector to my own humble plane of existence, where the trappings of terrestrial familiarity gradually coalesced around me once again. But everything now seemed just the slightest bit...skewed, as if the molecules of reality itself had been subtly rearranged by the force of my re-imaginings.As I surveyed my surroundings anew through dreamweaver's eyes, I was struck by the boundless possibilities now laid before me. No longer was I bound by the strictures of mundane physics or constraints of preconceived possibility. Every wish, whimsy and unbridled imagining was now within my grasp to actualize through sheer creative force of will. The entirecosmos was my lumenoietic canvas to rearrange and recompose in whatever ever-shifting patterns I could envision.With my first foray into the Metaversal Dreamrealms now complete, I felt a curious synergy of potential and uncertainty well up within me. Where would my burgeoning abilities as a Dreamweaver lead? What breathtaking or terrifying new emanations would I call forth into substantive existence from the morphaevic aethers? Perhaps it was best I didn't know just yet what audacious, improbable, fantastic, and wondrous feats of reconstructive meta-genesis awaited...。
A straw drift chamber spectrometer for studies of rare kaon decays
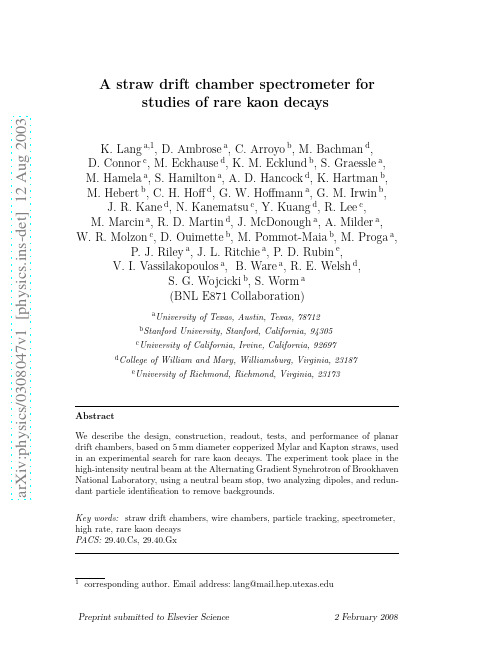
a rX iv:physics /03847v1[physics.ins -det]12Aug2003A straw drift chamber spectrometer for studies of rare kaon decays ng a ,1,D.Ambrose a ,C.Arroyo b ,M.Bachman d ,D.Connor c ,M.Eckhause d ,K.M.Ecklund b ,S.Graessle a ,M.Hamela a ,S.Hamilton a ,A.D.Hancock d ,K.Hartman b ,M.Hebert b ,C.H.Hoffd ,G.W.Hoffmann a ,G.M.Irwin b ,J.R.Kane d ,N.Kanematsu c ,Y.Kuang d ,R.Lee c ,M.Marcin a ,R.D.Martin d ,J.McDonough a ,der a ,W.R.Molzon c ,D.Ouimette b ,M.Pommot-Maia b ,M.Proga a ,P.J.Riley a ,J.L.Ritchie a ,P.D.Rubin e ,V.I.Vassilakopoulos a , B.Ware a ,R.E.Welsh d ,S.G.Wojcicki b ,S.Worm a (BNL E871Collaboration)a University of Texas,Austin,Texas,78712b Stanford University,Stanford,California,94305c University of California,Irvine,California,92697d College of William and Mary,Williamsburg,Virginia,23187e University of Richmond,Richmond,Virginia,231731IntroductionWe present a comprehensive description of a two-arm tracking system consist-ing of eight planar drift chambers based on5mm diameter straws designed, constructed,and operated in Alternating Gradient Synchrotron experiment 871at Brookhaven National Laboratory.Following a brief motivation for the experiment,we describe mechanical and electronics design considerations,im-portant construction details,and three years experience in operating the sys-tem.1.1Physics motivationDespite abundant experimental confirmations of the Standard Model of ele-mentary particles and interactions,the theory is generally perceived as incom-plete,or a low energy realization of a more general theory with a full symmetry at higher energy.There are indeed many basic questions to which answers will have to come from outside the current model.This is the main motivation for theoretical and experimental explorations beyond the Standard Model.In particular,leptonflavor violation occurs naturally in many extensions of the Standard Model;the process K L→µ±e∓is a sensitive probe of such viola-tion.In general,rare kaon decay experiments provided a promising avenue for discovering phenomena outside the Standard Model.The primary goal of experiment E871was to search for separate lepton number violation in the decay K L→µ±e∓.The experiment achieved a90%C.L.limit of4.7×10−12[1],thus probing new interactions in nature in the200-TeV mass scale.To achieve this sensitivity,the experiment ran with the very high beam intensities made possible by the Booster at the AGS.The experiment also made thefirst observation of the decay K L→e+e−,mea-suring a branching fraction of8.7+5.7−4.1×10−12based on four observed events[2]. Over6,000thousand K L→µ+µ−events were also observed,for a branching fraction of(7.19±0.17)×10−9,reducing the uncertainty for this decay mode by a factor of three compared to previous attempts[3].1.2Experimental setupTwo important experimental elements relevant to this work were the AGS high-intensity neutral beam and the E871two-arm spectrometer.A novel beam stop[4,5]was located in the upstream spectrometer magnet to improve downstream acceptance,tracking,and particle identification.Fig.1.BNL E871apparatus.The essential requirements on the spectrometer were good kinematic recon-struction and reliable,redundant particle identification,both to be accom-plished in a high rate environment.The spectrometer,shown in Figure1,was built in the B5beam line of the AGS.The neutral beam was produced by an intense primary24.1GeV/c beam of up to1.7×1013protons delivered in a1.6s spill onto a1.5-interaction-length platinum target.A system of sweep-ing magnets and collimators angled at3.75◦with respect to the proton beam direction formed a neutral beam with mostly neutrons and kaons at an ap-proximate solid angle of4×16mrad2.Particles emerging from the11m long evacuated decay volume were tracked and identified in the E871spectrometer. The main features of the apparatus were:•two magnets for redundant momentum measurement,tuned to form a“par-allel trigger”for two-body K L→µ±e∓decays(i.e.,charged particles trav-eling downstream of the second analyzing magnets triggered the apparatus only if they traveled nearly parallel to the neutral beam direction);•a beam-stop placed in thefirst magnet to absorb the neutral beam •redundantfinely-segmented fast straw drift chambers,followed by conven-tional drift chambers in regions of lower rate•redundant particle identification of muons and electrons•multi-level trigger with fast on-line reconstruction•fast custom-designed massively-parallel data acquisition system.The neutral beam was absorbed in a beam stop specially designed and tested for this configuration.The effective strengths of the magneticfields(+418and −216MeV/c)were set such that trajectories of two-body kaon decays emerged nearly parallel to the neutral beam direction downstream of the second mag-net[5].Such an arrangement simplified triggering and provided thefirst level rejection of the three-body decays.The intense primary proton beam produced roughly2×108K L decays per AGS spill,resulting in high hit rates in the upstream straw drift chambers. Additional rates in chambers resulted from leakage of low energy particles (mainly charged,but also neutrons and photons)from the beam-stop.The beam stop shielded the downstream part of the spectrometer,where rates were substantially reduced,thus minimizing the probability of pattern-recognition or particle-identification errors.Rejection of background due to common kaon decays depended crucially on precise,redundant and low-occupancy tracking.1.3Tracking system requirementsSince tracking resolution at low momentum is dominated by multiple Coulomb scattering,maximal background suppression required that the tracking sys-tem be low mass,efficient,redundant,fast(i.e.,possess low occupancy),and provide good position measurement.When designing experiment871the fol-lowing requirements for the tracking were thus imposed:(1)minimize multiple Coulomb scattering by limiting the amount of material;(2)provide tracking redundancy with low rate of wire failures and low celloccupancy;(3)provide high hit efficiency for minimum ionizing particles;(4)sustain good position resolution in high rate environment;(5)assure good vertex reconstruction of two-body decays.The corollary from the above list are general hardware features desired of the system:•high segmentation(i.e.,small cell size)•fast drift velocity gas•fast-timing electronics with short pulse tails•minimal cross-talk•mechanical robustness.We discuss the design and material choices made to accomplish the above requirements in the following sections.Beam Side View Off Beam Side ViewTop ViewFront View Fig.2.Front,top,and side views of an E871straw drift chamber.The amplifiers are shown in their nominal locations on the top and beam-out sides.2Mechanical designAs Figure 1illustrates,there were two straw stations between the decay tank and first spectrometer magnet,and two more between the magnets,followed by two conventional drift chamber stations downstream of the second magnet.An assembly drawing of a complete chamber module with x and y views is shown in Figure 2.2.1Choice of materialsThe technical possibility of making thin-wall tubes suggested the replacement of traditional drift chambers —with the electric field shaped by wires —by chambers based on a continuous cylindrical cathode surface,or “straw”.The name derives from the manufacturing process,which is similar to that of paper drinking straws.Thin,small straws with wall thicknesses of approximately 30µm and 5-mm diameter,were originally used in the vertex detectors of several high energy physics experiments at e +e −colliders [6],as well as in balloon flight experiments [7].They will be part of the ATLAS detector at the CERN Large Hadron Collider [8].Table 1summarizes the amounts of material in an individual tracking cham-ber,expressed in units of radiation length.The traditional 20µm gold-plated tungsten wire was used for the anode.As shown in the table,the total radia-tion thickness of a typical E871straw chamber was 0.21%of radiation length.Table1Radiation thickness of a straw drift chamber with3x and2y layers(in units of 10−4of X0).The straw intra-wall adhesive layer is accounted for by multiplying the number of straw layers(5)by120%.mean radiationthickness(cm)layers X0(cm)thickness(%X0)The thickness of conventional drift chambers was very similar.The materials most often used for straw walls are polyester(e.g.Mylar[10]) and polyimide(such as Kapton[11]).In our experience Kapton has the better dimensional stability of the two.Mylar straws installed for the1995run of E879showed signs of shrinkage over the course of the running period,making the individual straws appear twisted.This motivated our move to Kapton for rebuilt1996chambers;these chambers displayed no such effect.We attribute this difference in stability to the higher water content of Mylar relative to Kapton;Mylar became more brittle or“crinkly”with age—a sign of dehy-dration.Two metals are typically considered for cathode lamination:aluminum and copper.Another option is a carbon-doped substrate material with controlled resistivity.At the time of our construction,doped Polycarbonate sheets were available and we explored their use[12].However,the thinnest sheets available were15µm,and since Polycarbonate is mechanically weaker than Mylar,we did not pursue this option.The use of resistive substrate and copper cathodes was motivated by effects related to the aging/oxidizing of the aluminum cath-ode,as it had been suggested that a(semi-)conductive base material would remedy some of these problems.Similar comments can be made about Kapton. The thickness of Mylar and Kapton can be controlled with high precision;thin foils can be as little as2µm thick.Metalizing Mylar or Kapton up to1000˚A is easy and bining Mylar or Kapton with copper provided a strong straw base and led to less severe anode aging.Copper lamination was chosen because of its much better electrical aging properties than aluminum.Copper oxide is a semiconductor,which should lessen the possibility of cathodefield-emission effects,as opposed to aluminum dioxide,which is an insulator.Copper cathodes also have a relatively high work function,which provides some soft-photon absorption at the straw walls.2.2Physical design considerationsWe studied several possible planar cell patterns,trying to optimize effi-ciency and redundancy while minimizing radiation thickness and construction complexity.The configurations fall into three categories:close-packed(Fig-ure3(A)),rotated close-packed(Figure3(B)),and staggered(Figure3(C)). Close-pack arrangements are bonded together along the straw,forming self-supporting structures,while staggered designs require individually tensioned straws.The self-supporting nature of close-pack designs offers simplicity of design, since the array needs no external tension.Staggered straws offer greaterflex-ibility,with aflatter distribution of mean path lengths across the active area of the chamber,but require tension to keep from sagging.We studied the relative hit multiplicities of the various geometries via Monte Carlo simulation for the acceptance for tracks with angle ranges with respect to the neutral beam direction of−250to250,20to250,and20to150mr.To maintain high redundancy,we wanted to minimize the number of tracks for which two or fewer hits were observed per single-view tracking station.The simulation used the straw’s inner diameter of0.5cm as the size of the active area of each straw.Simulated E871tracks were projected onto the xz plane and any track crossing a straw’s active area was considered a hit.The studied geometries are shown in Figure3and summarized in Ta-ble 2.2.In thefirst design,a conventional3-view close-pack,thefinite straw-wall thickness allowed some2-hit tracks.The mean straw density was 1.97straws/cm/view.The second design,a“rotated”close pack,allowed no 2-hit tracks,while some tracks could have as many as6hits.The straw den-sity here was higher:2.27straws/cm/view.Both designs required straws to be glued together for mechanical stability.The third design was a staggered-straw geometry.This design presented a smoother gas path-length distribution and might have allowed replacement of individual straws.Moreover the position of the offset straw could be placed off-center in order to optimize the chamber for a desired angular acceptance.All geometries are shown for three measurement views.Table2.2compares the close-pack and rotated close-pack geometries to stag-(A) (B)Fig.3.(A)Close-pack,(B)rotated close-pack,and(C)staggered geometries.Tracksare vertical in all cases.gered straws at the same straw density.All configurations with2.27straws/cm/view appear to be hit-equivalent.The view densities used here should be consid-ered the two extremes.Only a skewed-stagger design,which provides plentyof straw-to-straw separation while still limiting the2-hit probability and view density,allows a continuous density adjustment.In summary,we found that the rotated close-pack and staggered designs with2.27straws/cm/view had similar hit characteristics.The mean numbers of hits were3.45and3.46respectively and neither had appreciable2-hit probability. Due to the unavoidable dead space between adjacent straws,the conventional close-pack design allowed about3%2-hit tracks.The tensioning required of staggered configurations would make chambers susceptible to“creep”,or relaxation of tension,which is expected from any plastic stretched beyond its glass transition point.In laboratory testing we observed this effect in our Mylar straws.While tensioning or gluing of thex(vertical-straw)view would have been unnecessary;y views,with horizon-tal straws,required either tension or structural support to maintain shape. Prototype chambers of both close pack and individually-tensioned,staggered straws were built and tested in cosmic-ray telescopes and in the AGS neutral beam,but the technical challenges associated with the individually-tensioned configuration led us to choose the conventional close pack design for the E871 spectrometer.Comparison of the hit-straw multiplicity,¯n,and distributions of the number of hit straws,n,close-pack(A)or rotated close-pack(B)geometry to staggered geometries of acceptances(C)-250–250mr,(D)20–250mr and(E)20–150mr.N.N.is the nearest-neighbor distance in cm.1.97straws/cmPercent n-hitsN.N.¯n n=234562.27straws/cmPercent n-hitsN.N.¯n n=23456SDC (y)DCH (y)Fig.4.Straw and conventional drift cell configurations for x and y views.Example tracks with(perfect)distances of closest approach(DOCAs)are shown.2.3Mechanical assemblyClose-pack cell configurations for the E871straw chambers are shown in com-parison to those of our conventional drift chambers in Figure4.For redun-Straw drift chamber characteristicsdancy in the momentum-measuring horizontal(xz)plane,the x-measuring views each had a redundant,third plane of drift cells.Each drift cell in the straw drift chambers consisted of a5-mm diameter cylindrical copper-plated Mylar or Kapton cathode[13,14]with a20-micron gold-plated tungsten sense wire[15]along its axis.The cylindrical cathodes were wound on precision man-drels,where they were built up from two25.4-µm layers of material bonded with a thin layer of4-8µm of adhesive.The inner layer had the0.1-µm cop-per layer vapor-deposited[16]on one side which faced the inside of the straw tube.The original straws in the experiment were made of Mylar.However,all but one of the chambers(called SDC4LY)were rebuilt with Kapton for the 1996run period.Cylindrical brass sleeves provided the mechanical support and electrical contact between the straws and the end-plates.Feedthroughs pass through the end-plates and into the sleeves.Individual straws were bonded into closely-packed arrays whose cross section is shown in Figure4.Sense wires were precisely positioned within the straws by molded Ultem[17]inserts,which also acted as gas feedthroughs.Ground contact between the copper cathodes and the aluminum chamber frames was made through gold-plated brass sleeves,which were press-fit onto the Ultem feedthroughs and silver-epoxied into the straws.More silver epoxy formed the electrical contact between the sleeves and the chamber frames,while also providing a gas seal.Figures5,6,7show a cross-section assembly drawing of the inserts,sleeves,and end-plate gas manifold.A more detailed end-plate assembly for one of the chambers,including amplifier-card mounting and gas connections,is shown in Figure8.The straw drift chamber characteristics are summarized in Table3.Straw tubeCollarWireholder / Ultem (Feedthru) V -grooveSense wireGas hole Brass pinEndplate ConductiveEpoxy Au -platedbrass sleeve Fig.5.Straw end assembly drawing.Fig.6.Standard and truncated ULTEM inserts,with plugsThe feedthrough assembly served a number of functions,including positioning,tensioning,and electrically isolating the anode wire from the end-plate.It also supported the electrical contact of the anode wire to the outside signal and high voltage,and provided gas flow into or out of the straw.A sketch of the end-plate and feedthrough assembly is shown in Figure 5.The wire was positioned at the center of the straw at the feedthrough with a precision of about 25µm using a V-shaped groove molded into the feedthrough near one end (Figure 7).Tension was maintained with a brass pin inserted and clamped into the slotted Ultem end opposite the V-groove.The pin diameter,about 50µm smaller than the inside diameter of the feedthrough,allowed the anode wire to extend along the pin.The wire was compressed between the brass pin and the (slotted)feedthrough wall with an Ultem ring,which slid over the outside of the feedthrough.The pin end of the feedthrough,the pin,and the compression ring extended outside the chamber end-plate.The Ultem material had a resistance of at least 100MΩthrough its wall thick-ness of 0.10inches.Gas was supplied to the straw tubes in parallel through a gas manifold milled into the end-plates.From the manifold,the gas entered the interior of the Ultem feedthrough via a pair of holes in the feedthrough walls.Fig.7.Wire-positioning V-grooveTable4Adhesives and encapsulants used in the E871straw chambersapplication name pot life cure time Since the straw assembly was essentially irreversible,we did a test assembly of the complete chamber frame,including end-plates and corner blocks.This was then dismantled for installation of straws.All straw-end pieces were de-burred and cleaned in an ultrasonic bath.Prior to assembly,holes with critical clearance,such as the Ultem clearance holes in the end-plates,were tested and cleaned by inserting the requisite gauge pin.Most gasfittings were installed in the frame pieces prior to assembly,since the assembled frame had insufficient clearance for installation.With the exception of thefirst chamber built(called SDC2R),which had a stainless steel frame,all frame pieces were aluminum. The straws,of Mylar or Kapton,were shipped with Tyvek[18]liners,in ei-ther PVC tubes or wooden crates.Thus thefirst step in the straw preparation was unpacking,de-lining,and inspection.Straws with visible inner surface irregularities(dents or lumps of glue)were rejected.Following a previous ex-periment[19],we constructed a simple straw cutting method consisting of a precision bored Al block,with a hole diameter about25µm larger than the outer diameter of straws,with a transverse slit cut through the bore.Cutting a straw only required insertion into the bore and sliding a razor into the slit. Straws were installed in each x and y view of each module separately.Be-fore the two views were combined,their straws were laminated,potted,and structurally glued to the view sub-frame.Straws were held together within an array through spot application of Epo-Tek301-2,a very liquid epoxy with an8-hour pot life and two day cure[23].After securing the straws preciselyin place with specially-made brackets mounted on the chamber frames,epoxy was applied in small(∼0.5mm-diameter)drops to4–6points along each tan-gent between straws.A gas-pressure dispenser was used,with tubular steel tips which allowed inner straws to be reached.At this point the two viewsfit loosely in their frames.Bolting and pinning the x and y views together completed and squared the module frame,making it possible to bond the straw arrays to the frames by applying Tra-Bond2143D epoxy[24]around each insert outside the chamber end-plates,but still inside the gas manifold.Electrical contact between the straw inner surface(via the gold-plated brass‘sleeve’)was made byfilling the gap between straw/sleeve end and inner end-plate surface with EPO-TEK410E silver-filled epoxy[23]. Although before installation individual straw assemblies were tested for leaks, the silver epoxy should form an additional gas seal;the2143D epoxy forms the manifold-straw seal.The frame assembly was completed by gluing down of the manifold cover with a bead of silver epoxy joining the aluminum cover to the end-plate andfilling the2143D epoxy around each ultem insert. Wires were installed after chamber assembly.We used20-µm,gold-plated tungsten wire.The wire was threaded through a blunted sewing needle,fed through the top insert,and lowered down the straw and out the bottom insert. To provide working clearance and hold the wire against the interior v-notch of the insert,the wire was deflected by a horizontal bar suspended below the chamber.A gold-plated brass pin was inserted into the top insert and clamped in place with an Ultem collar.Then tension was applied to the wire by clamping a70-g mass below the bottom insert and deflecting bar(some tension was lost to friction at the bar and at the Ultem notch).Wire tension was locked in by installing another brass pin in the bottom insert,again with a clamping Ultem collar.Because of the awkward position and close-pack clearances of this step,we designed a special tool for pin installation.Once we were satisfied with the wire installation,a drop of epoxy(301-2)at each end ensured the pins and wire ends stayed in place.We checked wire tensions by measuring the resonant frequency of a driven wire in a constant magneticfield.A custom device[28]performed the frequency measurement and provided the driving frequency,while a permanent magnet held near the wire center supplied thefield.Any wires not satisfying minimum tension or standofflimits were removed and replaced.3Readout electronicsSignals from the straw sense wires were amplified and discriminated on6-channel boards directly mounted on the straw end pins.The amplifier circuitFig.8.SDC chamber assembly.(Figure9)is a four-transistor design,decoupled from the high-power discrimi-nation stage by a1-to-2transformer[29].The gain was about20mV/µA with less than1.5%cross talk,and a typical operating threshold of1.5µA.The digitized signal was converted to a30-ns pulse and sent over a333-ft long, 500-ns delay line by a96-channel driver board,to be recorded by a6-bit, 1.75-ns least-count TDC.Since particle positions were determined from the drift times of ionization electrons,position resolution was directly affected by the precision of the drift-time measurement.The high particleflux expected in experiment871required a small cell size to minimize occupancy,with a high drift velocity gas for reduced dead time.The small cell size of the straw chambers called for tight packing of front-end channels,increasing the possibility of cross talk.Our plan to use a CF4drift gas,meanwhile,implied small pulse heights,requiring a high gain front-end amplifier.In addition,the front-end electronics were required to dovetail with existing equipment:32-channel gray code TDCs with1.875ns least count[33] coupled to the front-end electronics through97-conductor(32ECL channel) 500ns delay lines.Front-end components designed and built specifically for E871included6-channel amplifier/discriminator boards,which mounted di-rectly onto the chamber frames,and96-channel cable driver boards.A blockR2539+5.0VETEST R5512K R302.4KC14.1uF R1710K C17.1uFQ2BFT25R18680C222.2pF Q3BFT25C20.1uF R44680C12.1uF Xform-Q4BFT25R3222R1910R354.3K Q1BFT92R38330C3220pFD8BAV99CH0 INC18390pF R34100R20200R271K Xform+LOGIC 0R515.1+5VCVTH Xform+3144 13T1B PE8309C25.1uF R4343K C26.1uF12 138********1517U2B LOGIC 1CH0 OUTCH0 OUT C24.1uFR39B 220Xform-R39A220R525.1-5VCFig.9.Amplifier schematic for the straw drift chambers.A single amplifier and discriminator circuit is shown.500 ns DelayCablesTDCs32 channelCable Driver Boards 16 x 6 channels in 3 x 32 channels out on twist-n-flat cable Differential signalsAmp/Disc BoardsStraws ~8000 total6 straws/board Fig.10.E871straw chamber readoutdiagram of the straw chamber electronics setup is shown in Figure 10.Initial designs of the front end amplifier based on an integrated-circuit am-plifier proved costly (more than 12dollars per channel),while an ASIC-based design missed our time window.We therefore pursued a much less expensive option (less than five dollars per channel),based on a four-transistor discrete amplifier design.Our initial design,with high-voltage distribution and the analog amplifier circuit on the chamber-mounted board and the discriminator on a separate driver board,taught us that twist-n-flat cable made an excellentantenna.The discriminator stage was subsequently moved onto the chamber-mounted board.Thefinal6-channel amplifier/discriminator layout consisted of high-voltage, analog,and digital sections coexisting on one four-layer board.Copious grounding and surface-mounted components eased the tight space constraints, while SIP sockets facilitated mounting the cards directly onto the straw end pins.The bias high voltage and front-end amplifier low-voltage power,along with odd-and even-channel pulser signals for rudimentary electronics diagno-sis,were delivered by chamber-mounted busses.The digital half of the ampli-fier boards were supplied by a separate cable bus.3.1Early testingEarly bench tests with simulated straw pulses reported gains of(22.±1.2)mV/µA.Stable operation was achieved at thresholds as low as0.9µA.We found that DIP-package multi-channel transformers in our original amplifier design caused too much cross talk.After replacing these with single-channel packages,cross talk between adjacent channels was reduced to1.5%.The front-end amplifiers were also tested on prototype straw chambers.During cosmic ray tests the amplifiers again operated at thresholds below1µA,with overall straw efficiencies measured above97%.Single-wire position resolution for the cosmic ray tests was120µm for the Ar-ethane(50-50)gas-fill.3.2Full-system performanceDuring actual data taking for E871,the complete6500-channel straw cham-ber readout system performed nearly as well as in tests.Even in the noisy environment of a high energy physics experiment,all chambers operated at or below a1.3-µA threshold with a mean efficiency of96%.We experienced a significant board failure rate due to cold solder joints on the amplifier cards;a test stand was built to diagnose and repair these faults. The entire mechanical design and the front-end electronics system has sub-sequently been copied for use in the Fermilab FOCUS experiment(FNAL 831)[27].。
Free Decay of Turbulence and Breakdown of Self-Similarity

2
lowest wavenumber spectrum ∼ kn is independent of time. This leads in the Burgers decay to a new length-scale ℓ∗ (t) ≫ ℓ(t), with the k2 backtransfer spectrum dominating throughout the intermediate range kℓ∗ (t) ≫ 1 and kℓ(t) ≪ 1. An analogous “Gurbatov phenomenon” was found to occur in the Kraichnan passive scalar model4 . It is our purpose to present a similar theory of the breakdown of self-similarity for the 3D incompressible Navier-Stokes equations. Let us consider first the case in which the energy spectrum is dominated by the k4 backtransfer term at the lowest wavenumbers (as it will be if the initial spectrum has n ≥ 4). In this case, one may suppose that
where v (t) is the rms velocity fluctuation, ℓ(t) is the integral length-scale, and F (κ) is a dimensionless scaling function (see section 10.2 of Lesieur1 ). However, recent studies of two exactly soluble models—the Burgers equation3 and the Kraichnan white-noise passive scalar equation4 —have shown that such self-similarity does not always hold. In particular, Gurbatov et al.3 observed that decaying Burgers turbulence develops two distinct length-scales when the low wave number spectral exponent n lies in the range 1 < n < 2. The energy spectrum can then no longer be divided into just a low-wavenumber range kℓ(t) ≪ 1 with E (k, t) ∼ Akn and an inertial range kℓ(t) ≫ 1 with E (k, t) ∼ k−2 . Instead a new spectral range develops intermediate to these two with E (k, t) ∼ C (t)k2 , C (t) ∝ (t − t0 )1/2 ln−5/4 (t − t0 ). Since it was the first author of reference 3 who observed this state of affairs in Burgers decay5 , we call it the “Gurbatov phenomenon”. The explanation of this new range lies in the phenomenon of a k2 backtransfer for Burgers dynamics, the analogue of the k4 backtransfer discovered by Proudman and Reid6 in 1954 for the 3D Navier-Stokes equations. According to traditional beliefs, the backtransfer term in Burgers should be overwhelmed at low-wavenumbers by the original Akn spectrum, which, for 1 < n < 2, is asymptotically much the larger. This statement, however, ignores the fact that the coefficient C (t) of the backtransfer term is growing in time, while the coefficient A of the
BEC-BCS crossover, phase transitions and phase separation in polarized resonantly-paired su
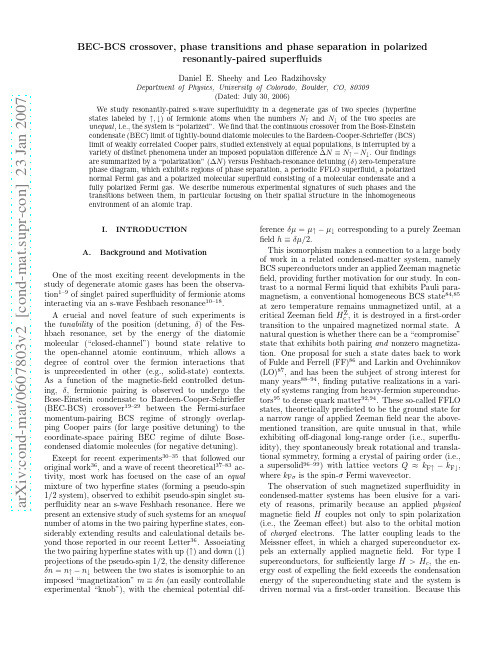
arXiv:cond-mat/0607803v2 [cond-mat.supr-con] 23 Jan 2007
Background and Motivation
One of the most in the study of degenerate atomic gases has been the observation1–9 of singlet paired superfluidity of fermionic atoms interacting via an s-wave Feshbach resonance10–18 . A crucial and novel feature of such experiments is the tunability of the position (detuning, δ ) of the Feshbach resonance, set by the energy of the diatomic molecular (“closed-channel”) bound state relative to the open-channel atomic continuum, which allows a degree of control over the fermion interactions that is unprecedented in other (e.g., solid-state) contexts. As a function of the magnetic-field controlled detuning, δ , fermionic pairing is observed to undergo the Bose-Einstein condensate to Bardeen-Cooper-Schrieffer (BEC-BCS) crossover19–29 between the Fermi-surface momentum-pairing BCS regime of strongly overlapping Cooper pairs (for large positive detuning) to the coordinate-space pairing BEC regime of dilute Bosecondensed diatomic molecules (for negative detuning). Except for recent experiments30–35 that followed our original work36 , and a wave of recent theoretical37–83 activity, most work has focused on the case of an equal mixture of two hyperfine states (forming a pseudo-spin 1/2 system), observed to exhibit pseudo-spin singlet superfluidity near an s-wave Feshbach resonance. Here we present an extensive study of such systems for an unequal number of atoms in the two pairing hyperfine states, considerably extending results and calculational details beyond those reported in our recent Letter36 . Associating the two pairing hyperfine states with up (↑) and down (↓) projections of the pseudo-spin 1/2, the density difference δn = n↑ − n↓ between the two states is isomorphic to an imposed “magnetization” m ≡ δn (an easily controllable experimental “knob”), with the chemical potential dif-
法布里珀罗基模共振英文

法布里珀罗基模共振英文The Fabryperot ResonanceOptics, the study of light and its properties, has been a subject of fascination for scientists and researchers for centuries. One of the fundamental phenomena in optics is the Fabry-Perot resonance, named after the French physicists Charles Fabry and Alfred Perot, who first described it in the late 19th century. This resonance effect has numerous applications in various fields, ranging from telecommunications to quantum physics, and its understanding is crucial in the development of advanced optical technologies.The Fabry-Perot resonance occurs when light is reflected multiple times between two parallel, partially reflective surfaces, known as mirrors. This creates a standing wave pattern within the cavity formed by the mirrors, where the light waves interfere constructively and destructively to produce a series of sharp peaks and valleys in the transmitted and reflected light intensity. The specific wavelengths at which the constructive interference occurs are known as the resonant wavelengths of the Fabry-Perot cavity.The resonant wavelengths of a Fabry-Perot cavity are determined bythe distance between the mirrors, the refractive index of the material within the cavity, and the wavelength of the incident light. When the optical path length, which is the product of the refractive index and the physical distance between the mirrors, is an integer multiple of the wavelength of the incident light, the light waves interfere constructively, resulting in a high-intensity transmission through the cavity. Conversely, when the optical path length is not an integer multiple of the wavelength, the light waves interfere destructively, leading to a low-intensity transmission.The sharpness of the resonant peaks in a Fabry-Perot cavity is determined by the reflectivity of the mirrors. Highly reflective mirrors result in a higher finesse, which is a measure of the ratio of the spacing between the resonant peaks to their width. This high finesse allows for the creation of narrow-linewidth, high-resolution optical filters and laser cavities, which are essential components in various optical systems.One of the key applications of the Fabry-Perot resonance is in the field of optical telecommunications. Fiber-optic communication systems often utilize Fabry-Perot filters to select specific wavelength channels for data transmission, enabling the efficient use of the available bandwidth in fiber-optic networks. These filters can be tuned by adjusting the mirror separation or the refractive index of the cavity, allowing for dynamic wavelength selection andreconfiguration of the communication system.Another important application of the Fabry-Perot resonance is in the field of laser technology. Fabry-Perot cavities are commonly used as the optical resonator in various types of lasers, providing the necessary feedback to sustain the lasing process. The high finesse of the Fabry-Perot cavity allows for the generation of highly monochromatic and coherent light, which is crucial for applications such as spectroscopy, interferometry, and precision metrology.In the realm of quantum physics, the Fabry-Perot resonance plays a crucial role in the study of cavity quantum electrodynamics (cQED). In cQED, atoms or other quantum systems are placed inside a Fabry-Perot cavity, where the strong interaction between the atoms and the confined electromagnetic field can lead to the observation of fascinating quantum phenomena, such as the Purcell effect, vacuum Rabi oscillations, and the generation of nonclassical states of light.Furthermore, the Fabry-Perot resonance has found applications in the field of optical sensing, where it is used to detect small changes in physical parameters, such as displacement, pressure, or temperature. The high sensitivity and stability of Fabry-Perot interferometers make them valuable tools in various sensing and measurement applications, ranging from seismic monitoring to the detection of gravitational waves.The Fabry-Perot resonance is a fundamental concept in optics that has enabled the development of numerous advanced optical technologies. Its versatility and importance in various fields of science and engineering have made it a subject of continuous research and innovation. As the field of optics continues to advance, the Fabry-Perot resonance will undoubtedly play an increasingly crucial role in shaping the future of optical systems and applications.。
不对称自由基反应英文

不对称自由基反应英文Asymmetric Radical Reactions: An Insight into Their Mechanism and Applications.Introduction.Asymmetric radical reactions have emerged as a powerful tool in organic synthesis, enabling the synthesis of chiral compounds with high enantiomeric purity. These reactions differ significantly from their symmetric counterparts, as they involve the generation and utilization of chiral radicals. These chiral radicals can undergo a range of reactions, including substitution, addition, and cyclization, leading to the formation of enantiomerically enriched products.Mechanism of Asymmetric Radical Reactions.The mechanism of asymmetric radical reactions typically involves three key steps: radical generation, chiralitytransfer, and radical termination.Radical Generation.The first step involves the generation of a radical species. This can be achieved through various methods, such as photolysis, thermal decomposition, or redox reactions. The generated radical can be chiral or achiral, depending on the starting materials and the conditions used.Chirality Transfer.The second step involves the transfer of chirality from a chiral auxiliary or catalyst to the radical species. This chirality transfer can occur through covalent or non-covalent interactions between the catalyst/auxiliary and the radical. The nature of these interactions determines the stereoselectivity of the reaction.Radical Termination.The final step involves the termination of the radicalspecies, leading to the formation of the desired product. This termination can occur through various mechanisms, such as coupling with another radical species, hydrogen atom abstraction, or disproportionation.Applications of Asymmetric Radical Reactions.Asymmetric radical reactions have found widespread applications in various fields of organic synthesis, including the synthesis of natural products, pharmaceuticals, and functional materials.Synthesis of Natural Products.Natural products often possess complex chiral structures, making their synthesis challenging. Asymmetric radical reactions have proven to be effective tools for the synthesis of such chiral natural products. For example, the use of chiral radicals generated from appropriate precursors has enabled the enantioselective synthesis of alkaloids, terpenes, and amino acids.Pharmaceutical Applications.The enantiomers of chiral drugs often differ significantly in their biological activities, making it crucial to control their enantiomeric purity. Asymmetric radical reactions can be used to synthesize enantiomerically enriched chiral drugs with high selectivity. This approach has been successfully applied to the synthesis of various drugs, including anti-inflammatory agents, anticancer agents, and antiviral agents.Functional Materials.Chiral materials possess unique physical and chemical properties that make them useful in various applications, such as displays, sensors, and catalysts. Asymmetricradical reactions can be used to synthesize chiral building blocks for the preparation of such materials. For instance, chiral polymers can be synthesized by utilizing asymmetric radical polymerization reactions, leading to the formation of materials with controlled chirality and tailored properties.Conclusion.Asymmetric radical reactions have emerged as powerful tools for the synthesis of enantiomerically enriched chiral compounds. Their unique mechanism, involving chirality transfer from a chiral catalyst/auxiliary to the radical species, enables high selectivity and enantiopurity in the product. The widespread applications of asymmetric radical reactions in organic synthesis, particularly in the synthesis of natural products, pharmaceuticals, and functional materials, highlight their importance in modern chemistry.Future Perspectives.Despite the significant progress made in the field of asymmetric radical reactions, there are still numerous challenges and opportunities for further exploration.Improving Selectivity and Efficiency.One of the key challenges in asymmetric radical reactions is achieving high selectivity and efficiency. While significant progress has been made in this area, there is still room for improvement. Future research could focus on developing new chiral catalysts/auxiliaries that can promote asymmetric radical reactions with higher selectivity and efficiency.Expanding the Scope of Reactions.Currently, the scope of asymmetric radical reactions is limited by the availability of suitable precursors and the reactivity of the generated radicals. Future research could aim to expand the scope of these reactions by developing new methods for generating radicals with desired functionalities and reactivities.Applications in Sustainable Chemistry.In the context of sustainable chemistry, asymmetric radical reactions offer an attractive alternative to traditional synthetic methods. By utilizing renewableresources and mild reaction conditions, asymmetric radical reactions could contribute to the development of more sustainable synthetic routes for the preparation of chiral compounds.Integration with Other Techniques.The integration of asymmetric radical reactions with other techniques, such as photocatalysis, electrochemistry, and microfluidics, could lead to the development of new and innovative synthetic methods. By combining the advantages of these techniques, it may be possible to achieve even higher selectivity, efficiency, and scalability in asymmetric radical reactions.In conclusion, asymmetric radical reactions have emerged as powerful tools for the synthesis of enantiomerically enriched chiral compounds. While significant progress has been made in this area, there are still numerous opportunities for further exploration and development. Future research in this field could lead tothe discovery of new and innovative synthetic methods with improved selectivity, efficiency, and sustainability.。
Hadronic B decays at BABAR

3/16/2002—3/23/2002, Les Arcs, France
Stanford Linear Accelerator Center, Stanford University, Stanford, CA 94309 Work supported in part by Department of Energy contract DE-AC03-76SF00515.
The decay B → J/ψ φ, which has not yet been observed, is explained with the occurrence of cc¯dd¯ rescattering into a cc¯ss¯ state.
The above decay modes have been studied at BABAR. The η is reconstructed in γγ or π+π−π0 final states and the η′ in the η(→ γγ)π+π− channel. The φ is reconstructed in the K+K− final state. Table 1 shows the preliminary results 1 obtained from the analysis of 50.9 fb−1 of data recorded at the Υ (4S) resonance [6].
were performed by the CLEO Collaboration [9]. At BABAR, the decay B → ηcK is exclusively reconstructed, with the ηc decaying in KSK±π±,
恒星撞击实验作文英语
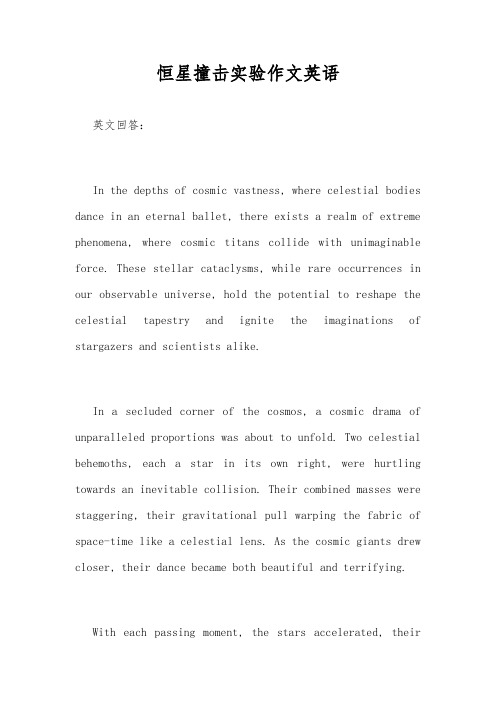
恒星撞击实验作文英语英文回答:In the depths of cosmic vastness, where celestial bodies dance in an eternal ballet, there exists a realm of extreme phenomena, where cosmic titans collide with unimaginable force. These stellar cataclysms, while rare occurrences in our observable universe, hold the potential to reshape the celestial tapestry and ignite the imaginations of stargazers and scientists alike.In a secluded corner of the cosmos, a cosmic drama of unparalleled proportions was about to unfold. Two celestial behemoths, each a star in its own right, were hurtling towards an inevitable collision. Their combined masses were staggering, their gravitational pull warping the fabric of space-time like a celestial lens. As the cosmic giants drew closer, their dance became both beautiful and terrifying.With each passing moment, the stars accelerated, theirsurfaces glowing with an incandescent brilliance. The celestial symphony reached its crescendo as the two cosmic leviathans finally collided in a blinding explosion of light and energy. The impact sent shockwaves rippling through the surrounding void, triggering a cosmic fireworks display that illuminated the celestial canvas.In the aftermath of the cataclysmic impact, a new celestial entity emerged from the cosmic debris. The fusion of the two stars had created a brilliant newborn star, its radiant glow illuminating the void where its predecessors once existed. The newly formed star, a testament to the chaotic beauty of the cosmos, embarked on its own journey through the galactic tapestry.The stellar collision experiment, as it came to be known, not only captivated the scientific community but also ignited the imaginations of poets and philosophers. It served as a cosmic parable about the transience of existence, the power of creation, and the indomitable spirit of the universe.As we gaze up at the night sky, shimmering with countless stars, let us remember the cosmic drama that played out in that distant corner of the cosmos. It is a reminder that even in the vast emptiness of space, life, death, and rebirth dance in an eternal cycle, shaping the grand narrative of the universe.中文回答:在宇宙浩瀚的深处,天体们在永恒的芭蕾中起舞,那里存在着极端的现象,宇宙巨兽以难以想象的力量相撞。
A Study of the Rare Decays $B^0to D_s^{()+} pi^-$ and $B^0to D_s^{()-} K^+$
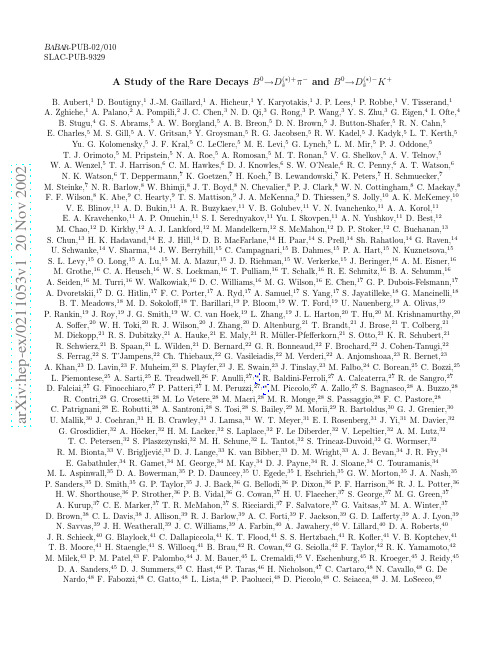
a r X i v :h e p -e x /0211053v 1 20 N o v 2002B A B A R -PUB-02/010SLAC-PUB-9329A Study of the Rare DecaysB 0→D (∗)+s π−and B 0→D (∗)−s K+B.Aubert,1D.Boutigny,1J.-M.Gaillard,1A.Hicheur,1Y.Karyotakis,1J.P.Lees,1P.Robbe,1V.Tisserand,1A.Zghiche,1A.Palano,2A.Pompili,2J.C.Chen,3N.D.Qi,3G.Rong,3P.Wang,3Y.S.Zhu,3G.Eigen,4I.Ofte,4B.Stugu,4G.S.Abrams,5A.W.Borgland,5A.B.Breon,5D.N.Brown,5J.Button-Shafer,5R.N.Cahn,5E.Charles,5M.S.Gill,5A.V.Gritsan,5Y.Groysman,5R.G.Jacobsen,5R.W.Kadel,5J.Kadyk,5L.T.Kerth,5Yu.G.Kolomensky,5J.F.Kral,5C.LeClerc,5M.E.Levi,5G.Lynch,5L.M.Mir,5P.J.Oddone,5T.J.Orimoto,5M.Pripstein,5N.A.Roe,5A.Romosan,5M.T.Ronan,5V.G.Shelkov,5A.V.Telnov,5W.A.Wenzel,5T.J.Harrison,6C.M.Hawkes,6D.J.Knowles,6S.W.O’Neale,6R.C.Penny,6A.T.Watson,6N.K.Watson,6T.Deppermann,7K.Goetzen,7H.Koch,7B.Lewandowski,7K.Peters,7H.Schmuecker,7M.Steinke,7N.R.Barlow,8W.Bhimji,8J.T.Boyd,8N.Chevalier,8P.J.Clark,8W.N.Cottingham,8C.Mackay,8F.F.Wilson,8K.Abe,9C.Hearty,9T.S.Mattison,9J.A.McKenna,9D.Thiessen,9S.Jolly,10A.K.McKemey,10V.E.Blinov,11A.D.Bukin,11A.R.Buzykaev,11V.B.Golubev,11V.N.Ivanchenko,11A.A.Korol,11E.A.Kravchenko,11A.P.Onuchin,11S.I.Serednyakov,11Yu.I.Skovpen,11A.N.Yushkov,11D.Best,12M.Chao,12D.Kirkby,nkford,12M.Mandelkern,12S.McMahon,12D.P.Stoker,12C.Buchanan,13S.Chun,13H.K.Hadavand,14E.J.Hill,14D.B.MacFarlane,14H.Paar,14S.Prell,14Sh.Rahatlou,14G.Raven,14U.Schwanke,14V.Sharma,14J.W.Berryhill,15C.Campagnari,15B.Dahmes,15P.A.Hart,15N.Kuznetsova,15S.L.Levy,15O.Long,15A.Lu,15M.A.Mazur,15J.D.Richman,15W.Verkerke,15J.Beringer,16A.M.Eisner,16M.Grothe,16C.A.Heusch,16W.S.Lockman,16T.Pulliam,16T.Schalk,16R.E.Schmitz,16B.A.Schumm,16A.Seiden,16M.Turri,16W.Walkowiak,16D.C.Williams,16M.G.Wilson,16E.Chen,17G.P.Dubois-Felsmann,17A.Dvoretskii,17D.G.Hitlin,17F.C.Porter,17A.Ryd,17A.Samuel,17S.Yang,17S.Jayatilleke,18G.Mancinelli,18B.T.Meadows,18M.D.Sokoloff,18T.Barillari,19P.Bloom,19W.T.Ford,19U.Nauenberg,19A.Olivas,19P.Rankin,19J.Roy,19J.G.Smith,19W.C.van Hoek,19L.Zhang,19J.L.Harton,20T.Hu,20M.Krishnamurthy,20A.Soffer,20W.H.Toki,20R.J.Wilson,20J.Zhang,20D.Altenburg,21T.Brandt,21J.Brose,21T.Colberg,21M.Dickopp,21R.S.Dubitzky,21A.Hauke,21E.Maly,21R.M¨u ller-Pfefferkorn,21S.Otto,21K.R.Schubert,21R.Schwierz,21B.Spaan,21L.Wilden,21D.Bernard,22G.R.Bonneaud,22F.Brochard,22J.Cohen-Tanugi,22S.Ferrag,22S.T’Jampens,22Ch.Thiebaux,22G.Vasileiadis,22M.Verderi,22A.Anjomshoaa,23R.Bernet,23A.Khan,vin,23F.Muheim,23S.Playfer,23J.E.Swain,23J.Tinslay,23M.Falbo,24C.Borean,25C.Bozzi,25L.Piemontese,25A.Sarti,25E.Treadwell,26F.Anulli,27,∗R.Baldini-Ferroli,27A.Calcaterra,27R.de Sangro,27D.Falciai,27G.Finocchiaro,27P.Patteri,27I.M.Peruzzi,27,∗M.Piccolo,27A.Zallo,27S.Bagnasco,28A.Buzzo,28R.Contri,28G.Crosetti,28M.Lo Vetere,28M.Macri,28M.R.Monge,28S.Passaggio,28F.C.Pastore,28C.Patrignani,28E.Robutti,28A.Santroni,28S.Tosi,28S.Bailey,29M.Morii,29R.Bartoldus,30G.J.Grenier,30U.Mallik,30J.Cochran,31H.B.Crawley,msa,31W.T.Meyer,31E.I.Rosenberg,31J.Yi,31M.Davier,32G.Grosdidier,32A.H¨o cker,cker,place,32F.Le Diberder,32V.Lepeltier,32A.M.Lutz,32T.C.Petersen,32S.Plaszczynski,32M.H.Schune,32L.Tantot,32S.Trincaz-Duvoid,32G.Wormser,32R.M.Bionta,33V.Brigljevi´c ,nge,33K.van Bibber,33D.M.Wright,33A.J.Bevan,34J.R.Fry,34E.Gabathuler,34R.Gamet,34M.George,34M.Kay,34D.J.Payne,34R.J.Sloane,34C.Touramanis,34M.L.Aspinwall,35D.A.Bowerman,35P.D.Dauncey,35U.Egede,35I.Eschrich,35G.W.Morton,35J.A.Nash,35P.Sanders,35D.Smith,35G.P.Taylor,35J.J.Back,36G.Bellodi,36P.Dixon,36P.F.Harrison,36R.J.L.Potter,36H.W.Shorthouse,36P.Strother,36P.B.Vidal,36G.Cowan,37H.U.Flaecher,37S.George,37M.G.Green,37A.Kurup,37C.E.Marker,37T.R.McMahon,37S.Ricciardi,37F.Salvatore,37G.Vaitsas,37M.A.Winter,37D.Brown,38C.L.Davis,38J.Allison,39R.J.Barlow,39A.C.Forti,39F.Jackson,fferty,39A.J.Lyon,39N.Savvas,39J.H.Weatherall,39J.C.Williams,39A.Farbin,40A.Jawahery,40V.Lillard,40D.A.Roberts,40J.R.Schieck,40G.Blaylock,41C.Dallapiccola,41K.T.Flood,41S.S.Hertzbach,41R.Kofler,41V.B.Koptchev,41T.B.Moore,41H.Staengle,41S.Willocq,41B.Brau,42R.Cowan,42G.Sciolla,42F.Taylor,42R.K.Yamamoto,ek,43P.M.Patel,43F.Palombo,44J.M.Bauer,45L.Cremaldi,45V.Eschenburg,45R.Kroeger,45J.Reidy,45D.A.Sanders,45D.J.Summers,45C.Hast,46P.Taras,46H.Nicholson,47C.Cartaro,48N.Cavallo,48G.De Nardo,48F.Fabozzi,48C.Gatto,48L.Lista,48P.Paolucci,48D.Piccolo,48C.Sciacca,48J.M.LoSecco,49J.R.G.Alsmiller,50T.A.Gabriel,50J.Brau,51R.Frey,51M.Iwasaki,51C.T.Potter,51N.B.Sinev,51D.Strom,51E.Torrence,51F.Colecchia,52A.Dorigo,52F.Galeazzi,52M.Margoni,52M.Morandin,52M.Posocco,52M.Rotondo,52F.Simonetto,52R.Stroili,52C.Voci,52M.Benayoun,53H.Briand,53J.Chauveau,53P.David,53 Ch.de la Vaissi`e re,53L.Del Buono,53O.Hamon,53Ph.Leruste,53J.Ocariz,53M.Pivk,53L.Roos,53J.Stark,53 P.F.Manfredi,54V.Re,54V.Speziali,54L.Gladney,55Q.H.Guo,55J.Panetta,55C.Angelini,56G.Batignani,56 S.Bettarini,56M.Bondioli,56F.Bucci,56G.Calderini,56E.Campagna,56M.Carpinelli,56F.Forti,56M.A.Giorgi,56 A.Lusiani,56G.Marchiori,56F.Martinez-Vidal,56M.Morganti,56N.Neri,56E.Paoloni,56M.Rama,56G.Rizzo,56F.Sandrelli,56G.Triggiani,56J.Walsh,56M.Haire,57D.Judd,57K.Paick,57L.Turnbull,57D.E.Wagoner,57 J.Albert,58N.Danielson,58P.Elmer,58C.Lu,58V.Miftakov,58J.Olsen,58S.F.Schaffner,58A.J.S.Smith,58A.Tumanov,58E.W.Varnes,58F.Bellini,59G.Cavoto,58,59D.del Re,59R.Faccini,14,59F.Ferrarotto,59F.Ferroni,59E.Leonardi,59M.A.Mazzoni,59S.Morganti,59G.Piredda,59F.Safai Tehrani,59M.Serra,59C.Voena,59S.Christ,60G.Wagner,60R.Waldi,60T.Adye,61N.De Groot,61B.Franek,61N.I.Geddes,61 G.P.Gopal,61S.M.Xella,61R.Aleksan,62S.Emery,62A.Gaidot,62P.-F.Giraud,62G.Hamel de Monchenault,62 W.Kozanecki,nger,62G.W.London,62B.Mayer,62G.Schott,62B.Serfass,62G.Vasseur,62Ch.Yeche,62 M.Zito,62M.V.Purohit,63A.W.Weidemann,63F.X.Yumiceva,63I.Adam,64D.Aston,64N.Berger,64A.M.Boyarski,64M.R.Convery,64D.P.Coupal,64D.Dong,64J.Dorfan,64W.Dunwoodie,64R.C.Field,64T.Glanzman,64S.J.Gowdy,64E.Grauges,64T.Haas,64T.Hadig,64V.Halyo,64T.Himel,64T.Hryn’ova,64 M.E.Huffer,64W.R.Innes,64C.P.Jessop,64M.H.Kelsey,64P.Kim,64M.L.Kocian,ngenegger,64 D.W.G.S.Leith,64S.Luitz,64V.Luth,64H.L.Lynch,64H.Marsiske,64S.Menke,64R.Messner,64D.R.Muller,64C.P.O’Grady,64V.E.Ozcan,64A.Perazzo,64M.Perl,64S.Petrak,64H.Quinn,64B.N.Ratcliff,64S.H.Robertson,64A.Roodman,64A.A.Salnikov,64T.Schietinger,64R.H.Schindler,64J.Schwiening,64G.Simi,64A.Snyder,64A.Soha,64S.M.Spanier,64J.Stelzer,64D.Su,64M.K.Sullivan,64H.A.Tanaka,64J.Va’vra,64S.R.Wagner,64M.Weaver,64A.J.R.Weinstein,64W.J.Wisniewski,64D.H.Wright,64C.C.Young,64P.R.Burchat,65C.H.Cheng,65T.I.Meyer,65C.Roat,65R.Henderson,66W.Bugg,67H.Cohn,67J.M.Izen,68 I.Kitayama,68X.C.Lou,68F.Bianchi,69M.Bona,69D.Gamba,69L.Bosisio,70G.Della Ricca,70S.Dittongo,70 nceri,70P.Poropat,70L.Vitale,70G.Vuagnin,70R.S.Panvini,71Sw.Banerjee,72C.M.Brown,72D.Fortin,72 P.D.Jackson,72R.Kowalewski,72J.M.Roney,72H.R.Band,73S.Dasu,73M.Datta,73A.M.Eichenbaum,73H.Hu,73J.R.Johnson,73R.Liu,73F.Di Lodovico,73A.Mohapatra,73Y.Pan,73R.Prepost,73I.J.Scott,73S.J.Sekula,73J.H.von Wimmersperg-Toeller,73J.Wu,73S.L.Wu,73Z.Yu,73and H.Neal74(The B A B A R Collaboration)1Laboratoire de Physique des Particules,F-74941Annecy-le-Vieux,France2Universit`a di Bari,Dipartimento di Fisica and INFN,I-70126Bari,Italy3Institute of High Energy Physics,Beijing100039,China4University of Bergen,Inst.of Physics,N-5007Bergen,Norway5Lawrence Berkeley National Laboratory and University of California,Berkeley,CA94720,USA6University of Birmingham,Birmingham,B152TT,United Kingdom7Ruhr Universit¨a t Bochum,Institut f¨u r Experimentalphysik1,D-44780Bochum,Germany8University of Bristol,Bristol BS81TL,United Kingdom9University of British Columbia,Vancouver,BC,Canada V6T1Z110Brunel University,Uxbridge,Middlesex UB83PH,United Kingdom11Budker Institute of Nuclear Physics,Novosibirsk630090,Russia12University of California at Irvine,Irvine,CA92697,USA13University of California at Los Angeles,Los Angeles,CA90024,USA14University of California at San Diego,La Jolla,CA92093,USA15University of California at Santa Barbara,Santa Barbara,CA93106,USA16University of California at Santa Cruz,Institute for Particle Physics,Santa Cruz,CA95064,USA17California Institute of Technology,Pasadena,CA91125,USA18University of Cincinnati,Cincinnati,OH45221,USA19University of Colorado,Boulder,CO80309,USA20Colorado State University,Fort Collins,CO80523,USA21Technische Universit¨a t Dresden,Institut f¨u r Kern-und Teilchenphysik,D-01062Dresden,Germany22Ecole Polytechnique,LLR,F-91128Palaiseau,France23University of Edinburgh,Edinburgh EH93JZ,United Kingdom24Elon University,Elon University,NC27244-2010,USA25Universit`a di Ferrara,Dipartimento di Fisica and INFN,I-44100Ferrara,Italy26Florida A&M University,Tallahassee,FL32307,USA27Laboratori Nazionali di Frascati dell’INFN,I-00044Frascati,Italy28Universit`a di Genova,Dipartimento di Fisica and INFN,I-16146Genova,Italy29Harvard University,Cambridge,MA02138,USA30University of Iowa,Iowa City,IA52242,USA31Iowa State University,Ames,IA50011-3160,USA32Laboratoire de l’Acc´e l´e rateur Lin´e aire,F-91898Orsay,France33Lawrence Livermore National Laboratory,Livermore,CA94550,USA34University of Liverpool,Liverpool L693BX,United Kingdom35University of London,Imperial College,London,SW72BW,United Kingdom36Queen Mary,University of London,E14NS,United Kingdom37University of London,Royal Holloway and Bedford New College,Egham,Surrey TW200EX,United Kingdom38University of Louisville,Louisville,KY40292,USA39University of Manchester,Manchester M139PL,United Kingdom40University of Maryland,College Park,MD20742,USA41University of Massachusetts,Amherst,MA01003,USA42Massachusetts Institute of Technology,Laboratory for Nuclear Science,Cambridge,MA02139,USA43McGill University,Montr´e al,QC,Canada H3A2T844Universit`a di Milano,Dipartimento di Fisica and INFN,I-20133Milano,Italy45University of Mississippi,University,MS38677,USA46Universit´e de Montr´e al,Laboratoire Ren´e J.A.L´e vesque,Montr´e al,QC,Canada H3C3J747Mount Holyoke College,South Hadley,MA01075,USA48Universit`a di Napoli Federico II,Dipartimento di Scienze Fisiche and INFN,I-80126,Napoli,Italy49University of Notre Dame,Notre Dame,IN46556,USA50Oak Ridge National Laboratory,Oak Ridge,TN37831,USA51University of Oregon,Eugene,OR97403,USA52Universit`a di Padova,Dipartimento di Fisica and INFN,I-35131Padova,Italy 53Universit´e s Paris VI et VII,Lab de Physique Nucl´e aire H.E.,F-75252Paris,France54Universit`a di Pavia,Dipartimento di Elettronica and INFN,I-27100Pavia,Italy55University of Pennsylvania,Philadelphia,PA19104,USA56Universit`a di Pisa,Scuola Normale Superiore and INFN,I-56010Pisa,Italy57Prairie View A&M University,Prairie View,TX77446,USA58Princeton University,Princeton,NJ08544,USA59Universit`a di Roma La Sapienza,Dipartimento di Fisica and INFN,I-00185Roma,Italy60Universit¨a t Rostock,D-18051Rostock,Germany61Rutherford Appleton Laboratory,Chilton,Didcot,Oxon,OX110QX,United Kingdom62DAPNIA,Commissariat`a l’Energie Atomique/Saclay,F-91191Gif-sur-Yvette,France63University of South Carolina,Columbia,SC29208,USA64Stanford Linear Accelerator Center,Stanford,CA94309,USA65Stanford University,Stanford,CA94305-4060,USA66TRIUMF,Vancouver,BC,Canada V6T2A367University of Tennessee,Knoxville,TN37996,USA68University of Texas at Dallas,Richardson,TX75083,USA69Universit`a di Torino,Dipartimento di Fisica Sperimentale and INFN,I-10125Torino,Italy 70Universit`a di Trieste,Dipartimento di Fisica and INFN,I-34127Trieste,Italy71Vanderbilt University,Nashville,TN37235,USA72University of Victoria,Victoria,BC,Canada V8W3P673University of Wisconsin,Madison,WI53706,USA74Yale University,New Haven,CT06511,USA(Dated:February7,2008)We report evidence for the decays B0→D+sπ−and B0→D−s K+and the results of a search for B0→D∗+π−and B0→D∗−s K+in a sample of84millionΥ(4S)decays into Bs4to demonstrate that the CKM mechanism is the correct explanation of this phenomenon.Several theoretically clean measurements of the angle βexist [3],but there is no such measurement of the two other angles αand γ.A theoretically clean measurement of sin(2β+γ)can be obtained from the study of the time evolution for B 0→D (∗)−π+[4]decays,which are already available in large samples at the B factories,and for the corre-sponding CKM-suppressed mode B 0→D (∗)+π−[5].FIG.1:The Feynman diagrams for the decays a)B 0→D (∗)−π+,b)B 0→D (∗)+π−,c)B 0→D (∗)+s π−,d)B 0→D (∗)−s K +.This measurement requires a knowledge of the ratio of the decay amplitudes R (∗)=|A (B 0→D (∗)+π−)/A (B 0→D (∗)−π+)|.Unfortunately a determination of |A (B 0→D (∗)+π−)|from a measurement of B (B 0→D (∗)+π−)is not possible with the currently available data sample due to the pres-ence of the large background fromB pairs collected in the years 1999-2002withthe B A B A R detector at the PEP-II asymmetric-energy B -factory [8].Since the B A B A R detector is described in de-tail elsewhere [9],only the components that are crucial to this analysis are summarized here.Charged particle tracking is provided by a five-layer silicon vertex tracker (SVT)and a 40-layer drift chamber (DCH).For charged-particle identification,ionization energy loss (dE/dx )in the DCH and SVT,and Cherenkov radiation detected in a ring-imaging device are used.Photons are identi-fied and measured using the electromagnetic calorimeter,which comprises 6580thallium-doped CsI crystals.These systems are mounted inside a 1.5T solenoidal supercon-ducting magnet.We use the GEANT [10]software to simulate interactions of particles traversing the B A B A R detector,taking into account the varying detector condi-tions and beam backgrounds.We select events with a minimum of four reconstructed charged tracks and a total measured energy greater than 4.5GeV,determined using all charged tracks and neutral clusters with energy above 30MeV.In order to reject con-tinuum background,the ratio of the second and zeroth order Fox-Wolfram moments [11]must be less than 0.5.So far,only upper limits have been reported for the modes studied here [12].Therefore the selection criteria are optimized to maximize the ratio of signal efficiency over the square-root of the expected number of back-ground events.Candidates for D +smesons are reconstructed in the modes D +s →φπ+,K 0S K +andK ∗0→K −π+.The K 0S candidates arereconstructed from two oppositely-charged tracks with an invariant mass 493<M π+π−<501MeV /c 2.All other tracks are required to originate from a vertex con-sistent with the e +e −interaction point.In order to iden-tify charged kaons,two selections are used:a pion veto with an efficiency of 95%for kaons and 20%for pions,and a tight kaon selection with an efficiency of 85%and 5%pion misidentification probability.Unless the tight selection is specified,the pion veto is always adopted.The φcandidates are reconstructed from two oppositely-charged kaons with an invariant mass 1009<M K +K −<1029MeV /c 2.TheK ∗0(φ)mesons in the D +s decaysare also utilized to reject backgrounds through the use of the helicity angle θH ,defined as the angle between one of the decay products of theK ∗0(φ)rest frame.Back-ground events are distributed uniformly in cos θH since they originate from random combinations,while signal events are distributed as cos 2θH .TheK ∗0π+,the K +in the reconstruction of D +s →K 0S K+or5 quired to pass the tight kaon identification criteria intro-duced above.Finally,the D+s candidates are required to havean invariant mass within10MeV/c2of the nominal value[13].We reconstruct D∗+s candidates in the mode D∗+s→D+sγ,by combining D+s and photon candidates. Photons that form aπ0candidate,with122<Mγγ< 147MeV/c2,in combination with any other photon with energy greater than70MeV are rejected.The mass dif-ference between the D∗+s and the D+s candidate is re-quired to be within14MeV/c2of the nominal value[13]. We combine D(∗)+scandidates with a track of opposite charge to form a B candidate,and assign the candidate to theq produc-tion.This source is suppressed based on event topology. We compute the angle(θT)between the thrust axis of the B meson candidate and the thrust axis of all other particles in the event.In the center-of-mass frame(c.m.), Bq pairs are produced back-to-back in the c.m.frame,which results in a|cosθT|distribution peaking at1.Based on the background level of each mode,|cosθT|is required to be smaller than a value that ranges between0.7and0.8.We further suppress backgrounds using a Fisher discriminant F constructed from the scalar sum of the c.m.momenta of all tracks and photons(excluding the B candidate de-cay products)flowing into9concentric cones centered on the thrust axis of the B candidate[14].The more spher-ical the event,the lower the value of F.We require F to be smaller than a threshold that varies from0.04to0.2 depending on the background level.We extract the signal using the kinematic variables m ES= m2i+p∗2i−E∗b,where E∗b is the beam energy in the c.m.frame, p∗i is the c.m.momentum of daughter particle i of the B meson candidate,and m i is the mass hypothesis for particle i.For signal events,m ES peaks at the B meson mass with a resolution of about2.5MeV/c2and∆E peaks near zero,indicating that the candidate system of particles has total energy consistent with the beam energy in the c.m.frame.The∆E signal band is defined by|∆E−5|<36MeV and within the band we define the events with m ES>5.27GeV/c2as the signal candidates. After the aforementioned selection,three classes of backgrounds remain.First,the amount of com-binatorial background in the signal region is esti-mated from the sideband of the m ES distribution∆E(GeV) FIG.2:The∆E distribution for B0→D+sπ−(top)and B0→D−sK+(bottom)candidates in data compared withthe distributions of the combinatorial background,estimated from the m ES sideband,the cross-contamination,estimatedfrom the M candD s sidebands,and the simulation of the signal, normalized to the observed yield.The insert shows the∆E distribution of the separate contributions to the cross con-tamination to the B0→D+sπ−signal as predicted by simula-tion.The reflection backgrounds are normalized to the known branching fractions[13],while the normalization of the charm-less background is arbitrary.which is described by a threshold function dN1−x2/E∗2bexp −ξ 1−x2/E∗2b ,characterized by the shape parameterξ[15].Second,B meson decays such asK∗0π+can constitute a background for the B0→D+sπ−mode if the pion in the D de-cay is misidentified as a kaon(reflection background). These backgrounds have the same m ES distributions as the signal but different distributions in∆E.The corresponding backgrounds for the B0→D−s K+mode (B0→D−K+,K∗+)have a branching fraction ten times smaller.Finally,rare B decays into the samefinal state,such as B0→K(∗)0K+K−(charmless back-ground),have the same m ES and∆E distributions as the B0→D+sπ−or B0→D−s K+signal.Figure2shows the ∆E distribution for the B0→D+sπ−and B0→D−s K+sig-nal and for various sources of background.The branching fraction of the charmless background is not well mea-sured;therefore we need to estimate the sum of the re-flection and charmless background(referred to as cross-contamination)directly with data.This is possible be-cause both of these background sources have aflat dis-tribution in the D+s candidate mass(M candD s)while the signal has a Gaussian distribution.6Possible contamination from B →D (∗)s X decays is de-termined with simulation and found to be negligible.The cross-contamination for the decays B 0→D ∗+s π−and B 0→D ∗−s K +is dominated by the reflection background,which we estimate from simulation.Cross-feed betweenB 0→D (∗)+s π−and B 0→D (∗)−s K +modes is estimated to be less than 1%.E v e n t s /2.5 M e V /c 2m ES (GeV/c 2)m ES (GeV/c 2)02.557.510012FIG.3:The m ES distributions for the B 0→D +s π−(topleft),B 0→D −s K +(top right),B 0→D ∗+s π−(bottom left),andB 0→D ∗−s K +(bottom right)candidates within the ∆E band in data after all selection requirements.The fits used to obtain the signal yield are described in the text.The contributionfrom each D +s mode is shown separately.Figure 3shows the m ES distribution in the ∆E signal band for each of the modes.We perform an unbinned maximum-likelihood fit to each m ES distribution with a threshold function to characterize the combinatorial background and a Gaussian distribution to describe the sum of the signal and cross-contamination contributions.The mean and the width of the Gaussian distribution are fixed to the values obtained in a copious B 0→D (∗)−π+control sample.For the B 0→D +s π−and B 0→D −s K +analyses,we obtain the threshold parameter ξfrom a fit to the distributions of m ES in data,after loosening the M cand D sand ∆E requirements.In the case of B 0→D ∗+sπ−and B 0→D ∗−s K +,due to the low background level,we use simulated events to estimate ξ.No fit is performed with the B 0→D ∗−s K +sample due to the small number of events.Whenever thereare enough events,we fit each D +s decay mode sepa-rately,as well as the combination of all modes.The cross-contamination is estimated by performing the samefit on the events in the data M candD ssidebands (4σ<|M cand D s −1968.6MeV /c 2|<8σ,where the resolution is σ=5MeV /c 2).The number of observed events,the background expectations,and the reconstruction efficien-cies estimated with simulated events are summarized in Table I.In the B 0→D +s π−(B 0→D −s K +)mode the fit yields a Gaussian contribution of 21.4±5.1(16.7±4.3)events and a combinatorial background of 7.8±1.7(3.5±1.3)events.The cross-contamination is estimated to be 3.7±2.4(2.7±1.9)events.The probability of the background to fluctuate to the observed number of events,taking into account both Poisson statistics and uncertainties in the background estimates,is 9.5×10−4(5.0×10−4).For a Gaussian distribution this would correspond to 3.3σ(3.5σ).Given the estimated reconstruction effi-ciencies we measure B (B 0→D +s π−)=(3.2±0.9)×10−5(B (B 0→D −s K +)=(3.2±1.0)×10−5),where the quoted error is statistical only.We also set the 90%C.L.lim-its B (B 0→D ∗+s π−)<4.1×10−5and B (B 0→D ∗−s K +)<2.5×10−5.The systematic errors are dominated by the 25%rela-tive uncertainty for B (D +s →φπ+).The uncertainties on the knowledge of the background come from uncertainties in the ξparameter,for the combinatorial background,and from the limited number of events in the M candDssidebands for the cross-contamination.They amount to 14%,16%,7%,and 36%of the measured branching frac-tions in the B 0→D +s π−,B 0→D −s K +,B 0→D ∗+s π−,and B 0→D ∗−s K +modes,respectively.The rest of the sys-tematic errors,which include the uncertainty on track-ing,K 0S reconstruction,and charged-kaon identification efficiencies,range between 11%and 14%depending on the mode.In conclusion,we report a 3.3σsignal for the b →utransition B 0→D +s π−and a 3.5σsignal for the decayB 0→D −s K +,and measure B (B 0→D +s π−)=(3.2±0.9(stat .)±1.0(syst .))×10−5,B (B 0→D −s K +)=(3.2±1.0(stat .)±1.0(syst .))×10−5.Since the dominant uncertainty comes from the knowl-edge of the D +s branching fractions we also computeB (B 0→D +s π−)×B (D +s →φπ+)=(1.13±0.33±0.21)×10−6and B (B 0→D −s K +)×B (D −s →φπ−)=(1.16±0.36±0.24)×10−6.The search for B 0→D ∗+s π−and B 0→D ∗−s K +yields the 90%C.L.upper limitsB (B 0→D ∗+sπ−)<4.1×10−5,B (B 0→D ∗−s K +)<2.5×10−5.We are grateful for the excellent luminosity and ma-chine conditions provided by our PEP-II colleagues,andfor the substantial dedicated effort from the comput-ing organizations that support B A B A R .The collabo-rating institutions wish to thank SLAC for its sup-port and kind hospitality.This work is supported by7 TABLE I:The number of signal candidates(N sigbox),the Gaussian yield(N gaus)and the combinatorial background(N comb) extracted from the likelihoodfit,the cross-contamination(N cross),the reconstruction efficiency(ε),the probability(P bckg)of the data being consistent with the backgroundfluctuating up to the level of the data in the absence of signal,the measured branching fraction(B),and the90%confidence-level upper limit.N gaus,N comb,and B are not available for modes with too few events.N cross is not reported if no event is found in the D+s mass sideband.B mode N gaus N cross B(10−5)(10−5)D+s→φπ+8.0±3.0<0.73.1±1.2K∗0K+9.2±3.42.9±1.83.5±1.951.9±0.612.38.3×10−2-all21.4±5.13.7±2.43.2±0.9±1.0B0→D∗+sπ−20.6±0.37.8--D+s→30.4±0.3 3.33.9×10−2<12D+s→K0S K+-<0.14-51.2±0.4N/A2.3×10−2<4.1D+s→φπ+5.8±2.61.1±1.22.4±1.3K∗0K+7.3±2.9<0.75.0±2.040.6±0.49.21.7×10−2-all16.7±4.32.7±1.93.2±1.0±1.0B0→D∗−sK+00.8±0.6 5.3--D+s→10.4±0.4 2.7--D+s →K0SK+-<0.14-21.6±0.8N/A0.48<2.5。
量子是一种玄学方法英语
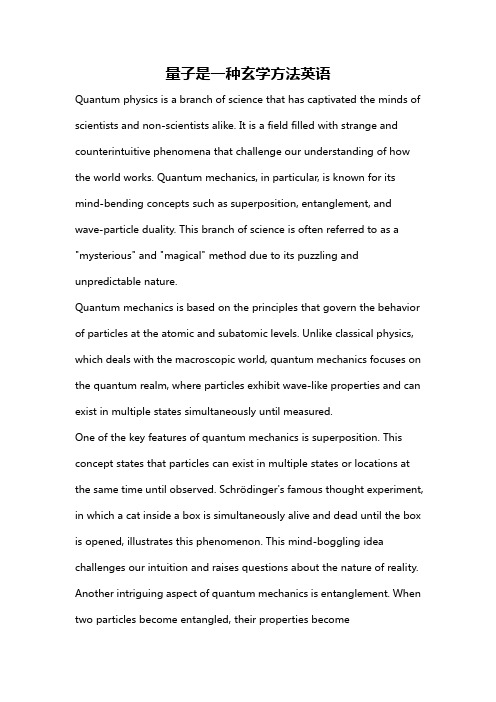
量子是一种玄学方法英语Quantum physics is a branch of science that has captivated the minds of scientists and non-scientists alike. It is a field filled with strange and counterintuitive phenomena that challenge our understanding of how the world works. Quantum mechanics, in particular, is known for its mind-bending concepts such as superposition, entanglement, and wave-particle duality. This branch of science is often referred to as a "mysterious" and "magical" method due to its puzzling and unpredictable nature.Quantum mechanics is based on the principles that govern the behavior of particles at the atomic and subatomic levels. Unlike classical physics, which deals with the macroscopic world, quantum mechanics focuses on the quantum realm, where particles exhibit wave-like properties and can exist in multiple states simultaneously until measured.One of the key features of quantum mechanics is superposition. This concept states that particles can exist in multiple states or locations at the same time until obser ved. Schrödinger's famous thought experiment, in which a cat inside a box is simultaneously alive and dead until the box is opened, illustrates this phenomenon. This mind-boggling idea challenges our intuition and raises questions about the nature of reality. Another intriguing aspect of quantum mechanics is entanglement. When two particles become entangled, their properties becomeinterdependent, regardless of the distance between them. This means that measuring the state of one particle instantaneously determines the state of the other, no matter how far apart they are. Einstein famously called this phenomenon "spooky action at a distance." The concept of entanglement has led to the development of quantum teleportation and quantum cryptography, which have the potential to revolutionize communication and computing.Furthermore, quantum mechanics challenges the classical concept of particles having definite properties. According to wave-particle duality, particles can behave as both waves and particles depending on the experimental setup. This means that particles can exhibit characteristics of both particles and waves simultaneously, adding to the mystery of quantum mechanics.Despite its success in explaining the behavior of atoms and subatomic particles, quantum mechanics is still not fully understood. It has been described as a "magical" and "mysterious" method due to its ability to produce unexpected and counterintuitive results. The probabilistic nature of quantum mechanics, where predictions are made based on the likelihood of outcomes rather than definitive results, adds to its enigmatic nature.The potential applications of quantum mechanics are vast. Quantum computers, currently in their infancy, have the potential to performcomplex calculations exponentially faster than classical computers. Quantum cryptography promises unbreakable encryption, ensuring secure communication in a world where digital security is crucial. Furthermore, quantum sensors have the ability to detect incredibly small changes in physical quantities, making them invaluable in fields like medicine, defense, and environmental monitoring.In conclusion, quantum mechanics is a field that continues to perplex and fascinate scientists and laypeople alike. Its counterintuitive concepts, such as superposition, entanglement, and wave-particle duality, make it appear as a mysterious and magical method. Despite its challenges, quantum mechanics holds immense potential for technological advancements and deeper understanding of the fundamental workings of the universe. As we continue to explore and unravel the mysteries of quantum physics, we embark on a thrilling journey into the unknown.。
Fuchsian polyhedra in Lorentzian space-forms
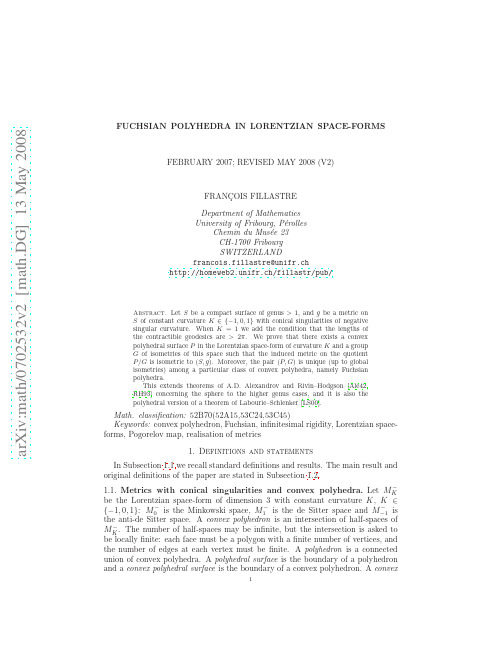
2
FUCHSIAN POLYHEDRA IN LORENTZIAN SPACE-FORMS
− (polyhedral) cone in MK is a convex polyhedral surface with only one vertex. In the de Sitter case, we will call polyhedral surface of hyperbolic type a polyhedral surface dual of a hyperbolic polyhedral surface (the definition of duality is recalled in Section 2). The sum of the angles between the edges on the faces of a space-like − − convex cone in MK (of hyperbolic type for M1 ) is strictly greater than 2π . A metric of curvature K with conical singularities of negative singular curvature on a compact surface S is a (Riemannian) metric of constant curvature K on S minus n points (x1 , . . . , xn ) such that the neighbourhood of each xi is isometric to − the induced metric on the neighbourhood of the vertex of a convex cone in MK . The xi are called the singular points. By definition the set of singular points is discrete, hence finite since the surface is compact. The angle αi around a singular point xi is the cone-angle at this point and the value (2π − αi ) is the singular curvature at xi . − Let P be a convex polyhedral surface in M1 of hyperbolic type homeomorphic to the sphere (note that the de Sitter space is not contractible). The induced metric on P is isometric to a spherical metric with conical singularities of negative singular curvature on the sphere. Moreover the lengths of the closed geodesics for this metric are > 2π , see [RH93]. The following theorem says that all the metrics of this kind can be obtained by such polyheion: 52B70(52A15,53C24,53C45) Keywords: convex polyhedron, Fuchsian, infinitesimal rigidity, Lorentzian spaceforms, Pogorelov map, realisation of metrics 1. Definitions and statements In Subsection 1.1 we recall standard definitions and results. The main result and original definitions of the paper are stated in Subsection 1.2.
the greeks assumed that长难句
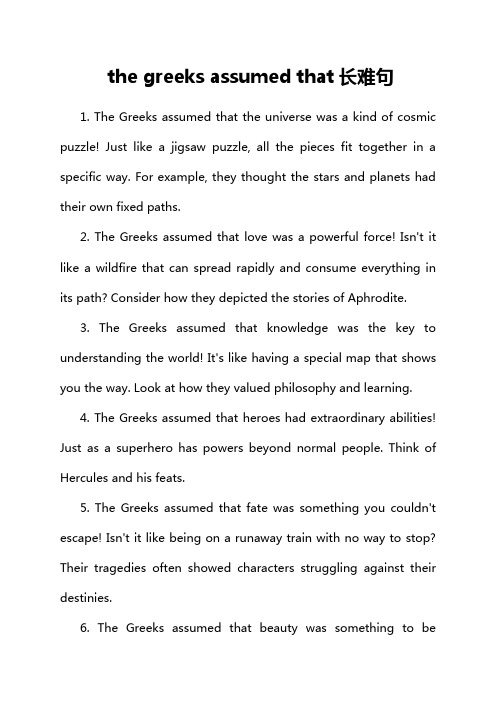
the greeks assumed that长难句1. The Greeks assumed that the universe was a kind of cosmic puzzle! Just like a jigsaw puzzle, all the pieces fit together in a specific way. For example, they thought the stars and planets had their own fixed paths.2. The Greeks assumed that love was a powerful force! Isn't it like a wildfire that can spread rapidly and consume everything in its path? Consider how they depicted the stories of Aphrodite.3. The Greeks assumed that knowledge was the key to understanding the world! It's like having a special map that shows you the way. Look at how they valued philosophy and learning.4. The Greeks assumed that heroes had extraordinary abilities! Just as a superhero has powers beyond normal people. Think of Hercules and his feats.5. The Greeks assumed that fate was something you couldn't escape! Isn't it like being on a runaway train with no way to stop? Their tragedies often showed characters struggling against their destinies.6. The Greeks assumed that beauty was something to beadmired! It's like a precious gem that catches your eye. Just look at their art and architecture.7. The Greeks assumed that courage was a virtue to be praised! Just like a shiny medal awarded to the bravest. Remember the tales of the Spartan warriors.8. The Greeks assumed that wisdom came with age! Isn't it like wine that gets better with time? Many of their wise men were older.9. The Greeks assumed that the gods had human-like qualities! It's like they were just super-powered versions of us. See how they portrayed the gods in their mythology.10. The Greeks assumed that music had the power to move the soul! Just like a gentle breeze that caresses your heart. Listen to their beautiful melodies.My view is that the Greeks' assumptions were really fascinating and have had a profound impact on our understanding of the world and human nature.。
stable diffusion 侧脸英文词

stable diffusion 侧脸英文词全文共10篇示例,供读者参考篇1Stable diffusion is a term that describes the movement of molecules from an area of high concentration to an area of low concentration in a steady and consistent manner. Imagine you have a balloon full of water and you poke a tiny hole in it. The water will slowly leak out of the hole and spread evenly throughout the balloon until it is all gone. That's kind of like stable diffusion!In our bodies, stable diffusion happens all the time. For example, when we breathe in oxygen, it diffuses from our lungs into our bloodstream so our cells can use it to make energy. And when we eat food, the nutrients in the food diffuse from our stomach and intestines into our blood so they can travel to different parts of our body.But stable diffusion isn't just important for our bodies. It's also important in nature. For example, when a drop of dye is added to a glass of water, it will slowly diffuse and spread out until the water is all colored. This process is called diffusion.In conclusion, stable diffusion is a natural process that helps things spread out evenly over time. It's like a slow and steady movement that happens all around us, both in our bodies and in nature. Pretty cool, huh?篇2Stable diffusion is super important. Do you know what it means? Let me tell you all about it!So, stable diffusion is when things spread out evenly. It's like when you mix chocolate powder into milk and it spreads out so that every sip tastes chocolatey. Cool, right?One example of stable diffusion is when you put a drop of food coloring into water. The color slowly spreads out until the whole glass of water is colored. It's like magic!Stable diffusion is also important in nature. Like, when plants take in nutrients from the soil, they use stable diffusion to spread the nutrients to all parts of the plant. This helps the plant grow big and strong.And did you know that stable diffusion is used in technology too? Yeah, like in batteries. The chemicals in batteries spread outevenly so that the battery can give power to things like toys and phones.So, stable diffusion is pretty awesome. It helps things spread out evenly and do their job properly. Next time you mix something or see colors blending together, remember to thank stable diffusion for making it happen!篇3Stable Diffusion- A Fantastic GameHello everyone! Today I want to tell you about a super cool game that I have been playing called Stable Diffusion. It's a game where you have to help little creatures move around a maze and reach their home safely. Sounds fun, right?The game starts off easy peasy, with just a few obstacles to dodge and some simple paths to follow. But as you progress through the levels, things start to get trickier and trickier. You have to think fast and plan your moves carefully to make sure the creatures don't get lost or trapped.One of the best parts of Stable Diffusion is all the different creatures you get to help. There are fluffy bunnies, speedy squirrels, and even a few mischievous raccoons. Each one hastheir own special abilities and challenges, so you have to be on your toes to keep them safe.The graphics in Stable Diffusion are super cute too. The colors are bright and the creatures are adorable. It's like playing in a cartoon world!I love playing Stable Diffusion because it's not only fun, but it also helps me think strategically and plan ahead. Plus, it's a great way to pass the time when I'm bored or waiting for my mom to finish cooking dinner.So if you're looking for a fun and challenging game to play, I definitely recommend giving Stable Diffusion a try. Trust me, you won't be able to stop playing once you start!篇4Stable DiffusionYo guys! Today we're gonna talk about this super cool thing called "stable diffusion". Sounds fancy, right? But don't worry,I'm gonna break it down for you in a way that even a little kid like me can understand.So, stable diffusion is basically about stuff moving around in a stable way. It's like when you drop a pebble into a pond andthe ripples spread out nice and evenly. That's stable diffusion in action!But stable diffusion isn't just about pebbles in ponds. It's also super important in things like science and technology. For example, when you spray air freshener in your room and the smell spreads out evenly, that's stable diffusion at work.Scientists and engineers use stable diffusion to make all kinds of cool stuff. Like when they design cars to be more fuel efficient, or figure out how to make medicines spread evenly in your body. It's like magic, but with science!One key thing to remember about stable diffusion is that it's all about keeping things nice and steady. No crazy jumps or sudden changes. Just a smooth, steady spread of whatever it is you're working with.So next time you see something spreading out nice and evenly, like when you pour milk into your cereal and it doesn't all clump together, remember that's stable diffusion doing its thing.And that, my friends, is stable diffusion in a nutshell. Pretty cool, huh? See, even us little kids can understand this stuff! Stay curious, stay awesome, and keep on spreading those ripples nice and steady. Catch you later!篇5Title: Stable diffusion -- Let's talk about it!Hi guys! Today I want to talk to you about something super cool called "stable diffusion." It sounds like a big, fancy word, but don't worry, I'll break it down for you in a way that's easy to understand.So, stable diffusion is basically when something spreads out evenly and stays in one place. It's kind of like when you put a drop of food coloring in a glass of water and watch it slowly spread out. The food coloring is diffusing, and if it spreads out evenly and stays in one place, that's stable diffusion.But why is stable diffusion important? Well, think about it like this - imagine if the food coloring just clumped together in one spot in the glass of water. That wouldn't be very helpful, right? Stable diffusion helps things like nutrients and oxygen move around in our bodies so that we stay healthy and strong.One cool example of stable diffusion is how our cells get the oxygen they need to function. The oxygen in our lungs diffuses into our blood, and then our blood carries it to all the different parts of our body. If the oxygen didn't diffuse evenly and staywhere it's needed, our cells wouldn't be able to do their job properly.So, the next time you hear the term "stable diffusion," remember that it's all about things spreading out evenly and staying put. It's a super important process that helps keep us alive and kicking! I hope you learned something new today. Until next time, stay curious and keep exploring the world around you. Bye, guys!篇6Title: Stable Diffusion - Side FaceHey guys! Today I'm going to talk to you about something super cool called stable diffusion. But wait, what's diffusion? Diffusion is when something spreads out and moves from one place to another. It's kind of like how your favorite smell can travel from the kitchen all the way to your room.Now, stable diffusion is a bit different. It's when something is able to spread out and move around, but it stays steady and doesn't change much. It's like when your best friend moves to a new school, but you still talk to them every day and keep your friendship strong.Imagine you have a bottle of your favorite perfume. When you open the bottle, the smell starts to spread out and fill the room. That's diffusion. But with stable diffusion, the smell stays in the room and doesn't disappear quickly. It hangs around and keeps making the room smell amazing.Scientists use stable diffusion to study how things move and spread in different environments. They can see how gases, liquids, and even heat move around and stay constant in one place. It's like a superpower that helps them understand the world better.So next time you walk into a room and smell something delicious cooking, remember that stable diffusion is at work. It's keeping that yummy smell in the air and making your mouth water.Stay curious, my friends, and keep exploring the world of science. Who knows what other cool things you'll discover with stable diffusion!篇7Stable diffusion sounds like a really fancy word, right? But don't worry, I'm here to explain it to you in a way that's easy tounderstand. So, stable diffusion basically means the process of something spreading out evenly and staying that way.Imagine you have a bowl of hot soup. If you leave it sitting on the counter for a while, the heat from the soup will spread out evenly throughout the bowl. This is an example of stable diffusion. The heat diffuses, or spreads out, so that the entire bowl of soup is warm, not just one part of it.Now, let's think about another example. Have you ever played with a drop of food coloring in a glass of water? When you first drop the color in, it stays in one spot. But as time goes on, the color starts to spread out until the entire glass of water is colored. This is also an example of stable diffusion.Stable diffusion happens all around us, even in our bodies! When we eat food, the nutrients from the food diffuse throughout our bodies, giving us the energy we need to run and play.So, the next time you hear the term stable diffusion, you'll know that it's just a fancy way of saying something is spreading out evenly and staying that way. Pretty cool, right?篇8Hey guys, do you know what stable diffusion means? Well, let me explain it to you in a fun and easy way!Stable diffusion is when stuff moves from one place to another in a smooth and steady way. It's like when you pour water on the ground and it spreads out evenly. Or when you blow bubbles and they float all around the room. That's stable diffusion!Stable diffusion is super important in science and nature. It helps things mix together, like when you stir your milk into your cereal. It also helps plants grow by making sure they get all the nutrients they need. Without stable diffusion, things would be all over the place and nothing would work right.So next time you see something spreading out or mixing together nicely, remember that's stable diffusion at work! It's like magic happening right in front of your eyes. Cool, right?I hope you guys learned something new about stable diffusion today. Remember, science is all around us and it's pretty awesome! Stay curious and keep exploring the world around you. See you next time!篇9Stable Diffusion is like when you put a yummy cake in the oven and the smell starts to spread all over the house. It's when something spreads out evenly and stays that way. Kind of like when you put a drop of food coloring in water and it slowly spreads out until the whole glass is the same color.But why does Stable Diffusion happen? Well, it's because of something called concentration. Concentration is like how much of something there is in a certain area. When there's more of something in one spot, it wants to spread out and even out with the other spots. It's kind of like when you have a big pile of candy and you want to share it with your friends so everyone has the same amount.Stable Diffusion is super important in science because it helps us understand how things move and spread. It's like when you mix a spoonful of sugar into your tea and watch it slowly dissolve. The sugar particles are moving around and spreading out until they're evenly mixed in.So next time you smell something delicious or watch something mix together, remember that's Stable Diffusion at work. It's like magic happening right before your eyes!篇10Today I want to tell you about something super cool! It's called stable diffusion. Well, it sounds like a fancy word but basically, it's all about how things spread out evenly.Imagine you have a big box of candies. If you pour them into a small bowl, all the candies might pile up on top of each other. But if you pour them into a bigger bowl, they spread out more evenly, right? That's kind of like stable diffusion!Stable diffusion happens when something spreads out in a stable and even way. It's like when you mix chocolate syrup into milk and it all blends together perfectly. Or when you pour a drop of food coloring into a glass of water and it slowly spreads out to color the whole glass.Stable diffusion is super important in lots of things. Like in plants, nutrients need to spread evenly from the roots to the leaves so the whole plant can grow healthy and strong. And in science, stable diffusion helps scientists understand how things move and mix together.So next time you see something spreading out evenly, remember stable diffusion! It's like magic how things can mix and spread out so perfectly. Cool, right?。
逻辑谬误英语作文乐队花车
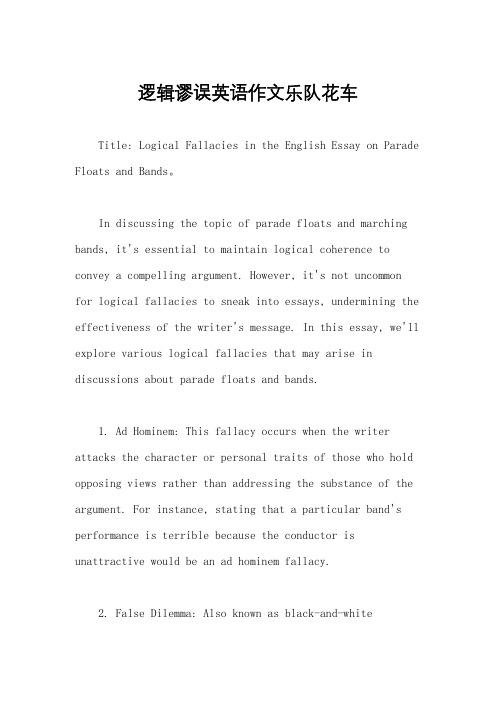
逻辑谬误英语作文乐队花车Title: Logical Fallacies in the English Essay on Parade Floats and Bands。
In discussing the topic of parade floats and marching bands, it's essential to maintain logical coherence to convey a compelling argument. However, it's not uncommonfor logical fallacies to sneak into essays, undermining the effectiveness of the writer's message. In this essay, we'll explore various logical fallacies that may arise in discussions about parade floats and bands.1. Ad Hominem: This fallacy occurs when the writer attacks the character or personal traits of those who hold opposing views rather than addressing the substance of the argument. For instance, stating that a particular band's performance is terrible because the conductor is unattractive would be an ad hominem fallacy.2. False Dilemma: Also known as black-and-whitethinking, this fallacy presents only two options when more exist. For example, arguing that parade floats are either entirely traditional or entirely modern neglects the possibility of a blend of both styles.3. Hasty Generalization: Drawing a conclusion based on insufficient or biased evidence characterizes this fallacy. An example would be asserting that all marching bands from a certain region lack talent based on a single performance.4. Appeal to Tradition: This fallacy argues that a practice or belief must be valid because "it's always been done that way." For instance, claiming that a particular float design is superior simply because it's been used for decades overlooks the potential for innovation.5. Appeal to Authority: Relying on the opinion of an authority figure rather than on evidence is an appeal to authority fallacy. If an essay asserts that a certain band is excellent because a celebrity said so without providing any further evidence, it commits this fallacy.6. Post Hoc Ergo Propter Hoc: Latin for "after this, therefore because of this," this fallacy assumes that because one event follows another, the first event caused the second. For instance, arguing that the presence of a specific float led to increased attendance at a parade without considering other factors would be a post hoc fallacy.7. Straw Man: This fallacy misrepresents an opponent's argument to make it easier to attack. For example, claiming that those who advocate for more modern parade floats wantto eliminate all traditional elements misrepresents the nuanced position of modernization advocates.8. Bandwagon Fallacy: This fallacy argues that because something is popular or widely believed, it must be correct. Claiming that a particular band is excellent solely because it has a large fan base commits this fallacy.9. Circular Reasoning: This occurs when the writer restates the argument rather than providing evidence to support it. For example, asserting that a parade float isthe best because it's the most popular without offering reasons why it's popular engages in circular reasoning.Avoiding these logical fallacies is crucial for constructing a persuasive and intellectually honest essay on parade floats and marching bands. By critically evaluating arguments and evidence, writers can create more compelling and convincing narratives that engage readers and promote meaningful dialogue.。
4.2荧光文献讲解
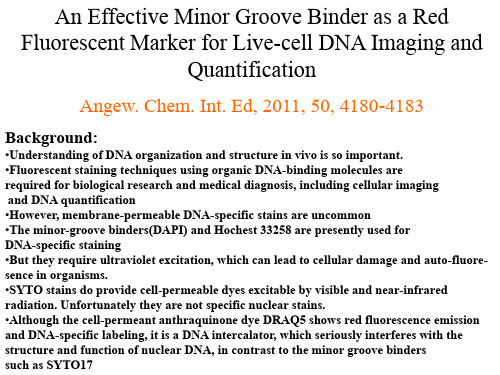
An Effective Minor Groove Binder as a Red Fluorescent Marker for Live-cell DNA Imaging and
Quantification
Angew. Chem. Int. Ed, 2011, 50, 4180-4183
•DEAB-TO-3 shows great fluorescence enhancement (97.3fold) when bound to native DNA and a distinct selectivity for dsDNA over total RNA (six times).
•DEAB-TO-3, as a red fluorescent live-cell-permeant DNA minor groove binder, is a promising candidate for highly sensitive DNA detection in vitro and nucleus-specific imaging and DNA quantification in vivo.
Conclusion:
1. a Cu(II) coumarin conjugate probe was developed that acted as a dual-response probe to HNO for both fluorescence and EPR detection.
Visualization of Nitroxyl in Living Cells by a Chelated
Copper(II) Coumarin Complex
- 1、下载文档前请自行甄别文档内容的完整性,平台不提供额外的编辑、内容补充、找答案等附加服务。
- 2、"仅部分预览"的文档,不可在线预览部分如存在完整性等问题,可反馈申请退款(可完整预览的文档不适用该条件!)。
- 3、如文档侵犯您的权益,请联系客服反馈,我们会尽快为您处理(人工客服工作时间:9:00-18:30)。
Fermi National Accelerator LaboratoryFERMILAB-Conf-99/192-TB Decays as a Probe of Spontaneous CP-Violation in SUSY ModelsOleg LebedevFor the CDF CollaborationVirginia TechBlacksburg,Virginia24061-0435Fermi National Accelerator LaboratoryP.O.Box500,Batavia,Illinois60510July1999Presented at SUSY’99,Fermilab,Batavia,Illinois,June14-19,1999Operated by Universities Research Association Inc.under Contract No.DE-AC02-76CH03000with the United States Department of EnergyB Decays as a Probe of Spontaneous CP-Violationin SUSY ModelsOleg LebedevPhysics Department, Virginia TechBlacksburg, VA 24061-0435Talk given at SUSY’99 (Fermilab, IL) andPHENO’99 (Madison, WI)Abstract: We consider phenomenological implications of susy models with spontaneously broken CP-symmetry. In particular, we analyze CP-asymmetries in B decays and find that the predictions of these models are vastly different from those of the SM. These features are common to NMSSM-like models with an arbitrary number of sterile superfields and the MSSM with broken R-parity.1.IntroductionOne of the most fundamental problems of particle physics is understanding the origin of CP-violation. In the Standard Model all CP-violating effects are described via Cabibbo-Kobayashi-Maskawa mechanism. In this approach, CP-violation originates from the quark mixing. However, in more general models, the origin of CP-violation can be quite different. For example, in multi Higgs Doublet Models, CP-symmetry can be broken spontaneously, that is to say, all CP-violating effects come entirely from complex phases in the HiggsVEV’s [1]. In supersymmetric models, this approach allows to significantly reduce the number offree parameters and to aviod excessive CP-violation inherent inlow energy supersymmetry [2]. Inthis work we discuss the CP phenomenology of these modelswhich is quite different from whatone expects in the Standard Modeland, thus, allows to distinguishbetween the two approaches.To begin with, we consider theNMSSM which has been shown tobe the simplest acceptable susyp-Conf-99-192-Tmodel allowing for spontaneous CP-violation [3]. The Higgs neutral components develop the following VEV’s:<H1>= v1 , <H2>= v2e i U,<N>= ne i[.These complex phases enter the left-right squark and gaugino-higgsino mixings, as well as the quark masses. By a universal phase redefinition of the right-handed quarks and squarks, the quark masses as well as the gauge interaction vertices can be made real. Thus the effect of the complex VEV’s shows up only in the Higgs (higgsino) sector and the left-right squark mixing.1 This can lead to observable CP-violating effects in the quark sector through loop effects involving super-particles. It has been shown [2] that with a favorable choice of the parameters the model can predict correct values of H and H'. In this work, we analyze the implications of the model for B physics.2. CP-violation in B decaysOne of the peculiar predictions of the Standard Model is the existence of the unitarity triangle. Information about the angles of this triangle can, for example, be 1 In general, the left-right squark mixing cannot be redefined to be real by a universal phase transformation due to the presence of A-terms.extracted from the following decays [4]:B \ K S a sin2E (1) B S+S-a sin2DB S U K S a sin2JIf the B L-B H lifetime difference and the tree-penguin interference effects can be neglected, the rate of the B decays into a final CP-eigenstate is described by a simple formula:*(B f i) e-*t (1 - sin2D isin 'mt) (2) Here D i are the angles of the unitarity triangle. In the SM this relation is not exact since the interference between the tree and penguin contributions can be significant. One has to invoke isospin and SU(3) relations among different processes to separate these contributions and to determine the angles of the unitarity triangle more precisely. However, in susy models with spontaneous CP-violation, relation (2) is much more precise. This happens due to the fact that the CP-violating contribution comes from the superpenguin diagram (Fig.1) which is strongly suppressed by heavy propagators and loop factors. As a result, the interference between the tree and superpenguin contributions is negligible. It is important to noteFig.1.Dominant CP-violating contri-bution to B decays.that, in our model, the angles ofthe unitarity triangle do not have aprocess independent meaning. Infact, they are defined by Eq.(2).The angles of the unitarity trianglecan be expressed in terms of thecomplex phases entering themixing and decay diagrams:sin2D i = sin(2I D + I M ) (3)As we have argued above, thedecay phase I D is vanishinglysmall. The mixing phase I M can beconsiderably larger since both theSM and susy contribute at oneloop level. In our model, there is anumber of large CP-conservingcontributions to 'B=2 operatorsuch as the SM box, charged Higgsbox, and the gluino and charginosuperboxes. All of thesecontributions interfere construc-tively. On the other hand, all butthe chargino CP-violating contri-butions (Fig.2) are suppressed bypowers of m b / m W [5]. In order tohave a sizeable CP-violation in thismodel, the existence of relativelylight susy particles is required.Fig.2. Dominant CP-violating contri-butions to the mixing (the cross on the scalar propagator denotes the L-R stop mixing).Moreover, the NEDM bound constraints the phases quite severely. As a result, large CP-violation cannot be accommodated within such models. From Eq.(3) one can determine typical values of the angles of the unitarity triangle. Since I M does not exceed 0.1 as required by the NEDM, the resulting triangle is either flat or very squashed and non-closing [6]. To be more quantitative, one can compare the prediction for the angle E with its SM value. In order for the CKM approach to be consistent, sin2E has to be greater than 0.4 (the CP asymmetry observed by CDF Collab. in B decays seems to agreeW ~b Qqq H ~b q q q q b b b W ~H ~W ~W ~H ~H ~W ~with this prediction). It isinteresting to see how large CP-Fig.3. The NEDM diagrams allowing toconstrain the phases entering the left-right squark and gaugino-higgsinomixings.violating phase is required in ourmodel to reproduce this result andwhether it is compatible with theNEDM bound. An illustrative plotshowing parameter space regionsallowed by sin2E 0.4 and theNEDM is given in Fig.4.Apparently, the NEDM andsin2E 0.4 cannot be accom-modated at the same time. It canalso be shown that the gapbetween the upper and lowerbounds does not decreaseconsiderably as one varies parameters of the model, i.e. tan E ,chargino mass, GIM cancellation parameter, etc. [5].Fig.4. Regions of the parameter space allowed by sin2E 0.4 and the NEDM.In fact, the same considerations are valid for a more general situation –when one includes more than one sterile superfield. Even though there are many more phases in the scalar VEV’s, these phases enter the NEDM and neutral meson mixing diagrams in the same combination. Thus, if the SM prediction for sin2E gets confirmed, a whole class of susy models with spontaneous CP-violation will be ruled out. Another “next-to-minimal” mo-del admitting spontaneous CPV is the MSSM with broken R-parity.In general, the sneutrinos can develop complex VEV’s and, in this case, one effectively deals with a 5 Higgs Doublet Model.Besides that, the FCNC areW ~H ~g~L d Rd L d Rd 00.20.40.60.81 100225475600 GeV δsin 350q m ~NEDM S K B ψ→,0z = ,5.0=ζ ,1tan =β 100~=W m GeVFig.5. R-breaking contributions toB \ K S decay and B-B mixing.generated at the tree level. Thediagrams relevant to ourconsiderations are given in Fig.5.They are required not to exceedtheir SM counterparts. Since weare interested in CP-violation inthe down type quark sector, wemay neglect the effect of complexsneutrino VEV’s due to the strictconstraints on the absolute valuesof the latter (~250 keV) [7] and O 'couplings.2 Then, in order to produce CP-violation, one would have to make left-right sparticle mixing insertions on the propagators. Due to the chiral structure of the R-breaking interactions, at least two such insertions are required on each propagator. As a result, CP-violating diagrams get suppressed by the fermion mass squared and can be neglected as compared to their CP-conserving counterparts.This means that large CP-violation in the B sector (sin2E 0.4) cannot be produced and the model suffers from the same shortcoming as the ones described previously. To summarize, the SM and susy models with spontaneous CP-violation lead to vastly different phenomenology in the B sector.The latter will be ruled out 3 if the SM prediction, sin2E 0.4, is experimentally confirmed. The author is grateful to the Fermilab Theory Group where part of this work was done. 2 Via the O 'LQD interactions, the sneutrino VEV’s may produce non-universal phases in the down type quark mass matrix. The effect,however, is small as explained above.3 We do not consider the possibility of accidentalcancellations among contributions to the NEDM.The conclusions would not hold if such cancel-lations occurred.bccR d ~sbsccLe ~b b d dLν~References[1] T.D. Lee, Phys. Rev. D 8 (1973) 1226.[2] A. Pomarol, Phys. Rev. D 47 (1993) 273.[3] A.T. Davies, C. D. Froggatt, A. Usai, in: Proc. of the Inter. Europhys. Conf. On HEP, Jerusalem (1997); hep-ph/9902476.[4] I.I. Bigi, F. Gabbiani, Nucl. Phys. B 352 (1991) 309.[5] O. Lebedev, hep-ph/9905216.[6] O. Lebedev, Phys. Lett. B 452 (1999) 294; hep-ph/9812501.[7] V. Bednyakov, A. Faessler, S. Kovalenko, Phys. Lett. B 442 (1998) 203; hep-ph/9904414.。