2015-nature-Square ice in graphene nanocapillaries
219401819_双水相提取金银花叶总黄酮工艺优化及其抗氧化活性
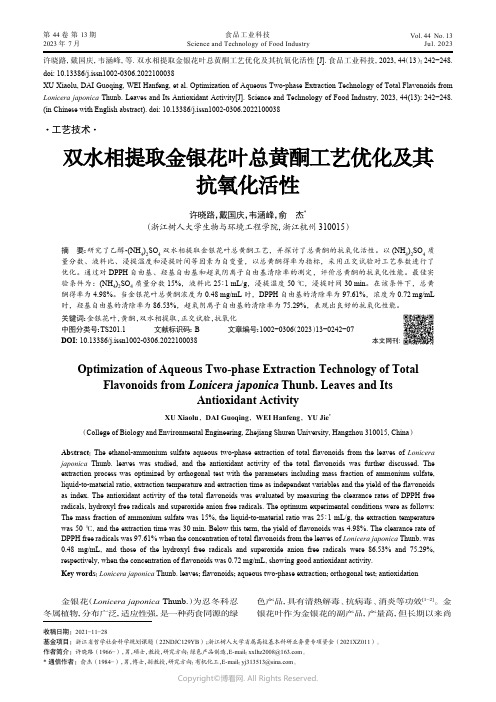
许晓路,戴国庆,韦涵峰,等. 双水相提取金银花叶总黄酮工艺优化及其抗氧化活性[J]. 食品工业科技,2023,44(13):242−248.doi: 10.13386/j.issn1002-0306.2022100038XU Xiaolu, DAI Guoqing, WEI Hanfeng, et al. Optimization of Aqueous Two-phase Extraction Technology of Total Flavonoids from Lonicera japonica Thunb. Leaves and Its Antioxidant Activity[J]. Science and Technology of Food Industry, 2023, 44(13): 242−248.(in Chinese with English abstract). doi: 10.13386/j.issn1002-0306.2022100038· 工艺技术 ·双水相提取金银花叶总黄酮工艺优化及其抗氧化活性许晓路,戴国庆,韦涵峰,俞 杰*(浙江树人大学生物与环境工程学院,浙江杭州 310015)摘 要:研究了乙醇-(NH 4)2SO 4双水相提取金银花叶总黄酮工艺,并探讨了总黄酮的抗氧化活性。
以(NH 4)2SO 4质量分数、液料比、浸提温度和浸提时间等因素为自变量,以总黄酮得率为指标,采用正交试验对工艺参数进行了优化。
通过对DPPH 自由基、羟基自由基和超氧阴离子自由基清除率的测定,评价总黄酮的抗氧化性能。
最佳实验条件为:(NH 4)2SO 4质量分数15%,液料比25:1 mL/g ,浸提温度50 ℃,浸提时间30 min 。
在该条件下,总黄酮得率为4.98%。
当金银花叶总黄酮浓度为0.48 mg/mL 时,DPPH 自由基的清除率为97.61%,浓度为0.72 mg/mL 时,羟基自由基的清除率为86.53%,超氧阴离子自由基的清除率为75.29%,表现出良好的抗氧化性能。
两级蓄冷跨临界压缩CO2混合工质储能系统特性分析

第 54 卷第 10 期2023 年 10 月中南大学学报(自然科学版)Journal of Central South University (Science and Technology)V ol.54 No.10Oct. 2023两级蓄冷跨临界压缩CO 2混合工质储能系统特性分析赵攀,吴汶泽,许文盼,刘艾杰,王江峰(西安交通大学 能源与动力工程学院,陕西 西安,710049)摘要:为了解决高压CO 2在高环境温度下难以冷凝的问题,提出两级蓄冷跨临界压缩CO 2混合工质储能系统。
采用CO 2与低沸点有机工质混合的方法提高工质的冷凝温度,同时,利用两级甲醇蓄冷实现系统内部冷能循环利用。
从环境性、临界温度、温度滑移、可混合性等方面确定合适的CO 2混合工质及其组分质量分数范围。
建立储能系统的热力学分析模型,探究节流压力、高压储液罐压力、有机工质质量分数等关键参数对系统性能的影响规律,并研究系统内部能量流动规律,得到主要部件的㶲损失。
研究结果表明:随着有机工质质量分数的增加,蓄冷介质温度增加,系统安全性提高;与纯CO 2工质相比,系统的充放电效率和能量密度略有降低;CO 2/R32混合工质的充放电效率最高为62.29%,CO 2/pentane 混合工质的能量密度最高为21.37 kW ∙h/m 3。
关键词:压缩CO 2储能;CO 2混合工质;热力学分析;敏感性分析;有机工质中图分类号:TK02 文献标志码:A 开放科学(资源服务)标识码(OSID)文章编号:1672-7207(2023)10-4150-13Performance analysis of a transcritical compressed CO 2-based mixture energy storage system with two-stage cold energy storageZHAO Pan, WU Wenze, XU Wenpan, LIU Aijie, WANG Jiangfeng(School of Energy and Power Engineering, Xi'an Jiaotong University, Xi'an 710049, China)Abstract: In order to solve the problem of difficult condensation of high-pressure CO 2 in higher ambient temperature, a transcritical compressed CO 2-based mixture energy storage system with two-stage cold energy storage was proposed. The CO 2-based mixture via blending CO 2 and organic working medium with low boiling point was selected to improve the condensation temperature. Meanwhile, a two-stage cold energy storage system via methanol was employed to store and recycle the cold energy. Firstly, the feasible CO 2-based mixtures and the related mass fraction ranges of organic working mediums were determined by considering the environment, critical temperature, temperature glide and miscibility. Then, the system thermodynamic models were established, and the effect of severalkey parameters on system performance was explored, such as throttling pressure, high-pressure storage tank pressure收稿日期: 2022 −10 −10; 修回日期: 2023 −02 −27基金项目(Foundation item):国家自然科学基金资助项目(51876152) (Project(51876152) supported by the National Natural ScienceFoundation of China)通信作者:赵攀,博士,教授,从事压缩气体储能技术基础与应用研究;E-mail :*****************DOI: 10.11817/j.issn.1672-7207.2023.10.032引用格式: 赵攀, 吴汶泽, 许文盼, 等. 两级蓄冷跨临界压缩CO 2混合工质储能系统特性分析[J]. 中南大学学报(自然科学版), 2023, 54(10): 4150−4162.Citation: ZHAO Pan, WU Wenze, XU Wenpan, et al. Performance analysis of a transcritical compressed CO 2-based mixture energy storage system with two-stage cold energy storage[J]. Journal of Central South University(Science and Technology), 2023, 54(10): 4150−4162.第 10 期赵攀,等:两级蓄冷跨临界压缩CO 2混合工质储能系统特性分析and mass fraction of organic working medium. At the same time, the system internal energy flow was analyzed, and the exergy destruction distribution of main components was obtained. The results show that the cold energy storage medium temperature increases and the system safety is enhanced with the increase of mass fraction of the organic working mediums. Compared with pure CO 2, the round-trip efficiency and energy density of CO 2-based mixture decrease slightly. The round-trip efficiency of CO 2/R32 mixture has the maximum value of 62.29%. The energy density with CO 2/pentane mixture reaches the maximum value of 21.37 kW ∙h/m 3.Key words: compressed CO 2 energy storage; CO 2-based mixture; thermodynamic analysis; sensitivity analysis; organic working medium能源是人类社会的物质基础,是经济发展的重要保障。
2015年度高校科学研究优秀成果奖公布
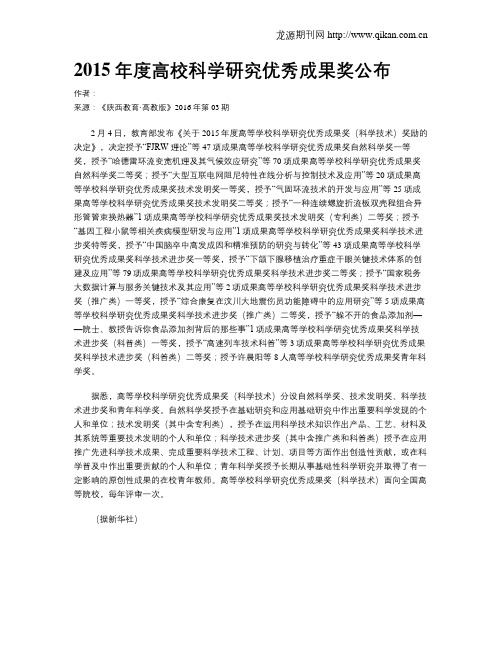
龙源期刊网 2015年度高校科学研究优秀成果奖公布作者:来源:《陕西教育·高教版》2016年第03期2月4日,教育部发布《关于2015年度高等学校科学研究优秀成果奖(科学技术)奖励的决定》,决定授予“FJRW 理论”等47项成果高等学校科学研究优秀成果奖自然科学奖一等奖,授予“哈德雷环流变宽机理及其气候效应研究”等70项成果高等学校科学研究优秀成果奖自然科学奖二等奖;授予“大型互联电网阻尼特性在线分析与控制技术及应用”等20项成果高等学校科学研究优秀成果奖技术发明奖一等奖,授予“气固环流技术的开发与应用”等25项成果高等学校科学研究优秀成果奖技术发明奖二等奖;授予“一种连续螺旋折流板双壳程组合异形管管束换热器”1项成果高等学校科学研究优秀成果奖技术发明奖(专利类)二等奖;授予“基因工程小鼠等相关疾病模型研发与应用”1项成果高等学校科学研究优秀成果奖科学技术进步奖特等奖,授予“中国脑卒中高发成因和精准预防的研究与转化”等43项成果高等学校科学研究优秀成果奖科学技术进步奖一等奖,授予“下颌下腺移植治疗重症干眼关键技术体系的创建及应用”等79项成果高等学校科学研究优秀成果奖科学技术进步奖二等奖;授予“国家税务大数据计算与服务关键技术及其应用”等2项成果高等学校科学研究优秀成果奖科学技术进步奖(推广类)一等奖,授予“综合康复在汶川大地震伤员功能障碍中的应用研究”等5项成果高等学校科学研究优秀成果奖科学技术进步奖(推广类)二等奖,授予“躲不开的食品添加剂——院士、教授告诉你食品添加剂背后的那些事”1项成果高等学校科学研究优秀成果奖科学技术进步奖(科普类)一等奖,授予“高速列车技术科普”等3项成果高等学校科学研究优秀成果奖科学技术进步奖(科普类)二等奖;授予许晨阳等8人高等学校科学研究优秀成果奖青年科学奖。
据悉,高等学校科学研究优秀成果奖(科学技术)分设自然科学奖、技术发明奖、科学技术进步奖和青年科学奖。
吸附工艺脱除柴油中芳烃的研究进展
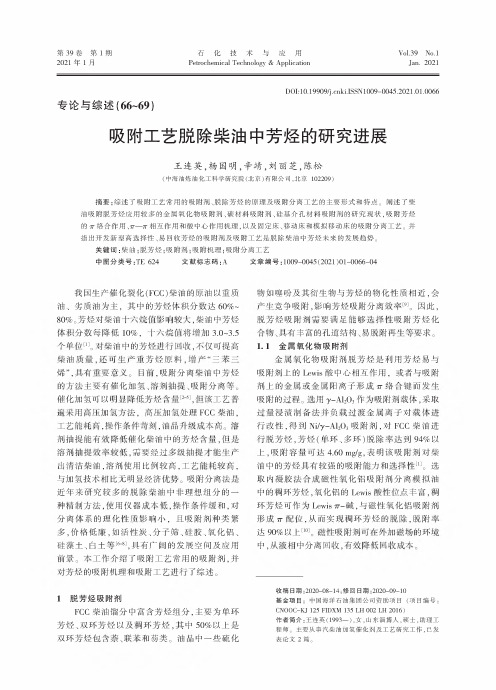
第39卷第1期2021年1月石化技术与应用Petrochemical Technology&ApplicationVol.39No.1Jan.2021DOI:10.19909/ki.ISSN1009-0045.2021.01.0066专论与综述(66-69)吸附工艺脱除柴油中芳?的研究进展王连英,杨国明,辛靖,刘丽芝,陈松(中海油炼油化工科学研究院(北京)有限公司,北京102209)摘要:综述了吸附工艺常用的吸附剂、脱除芳S的原理及吸附分离工艺的主要形式和特点。
阐述了柴油吸附脱芳S应用较多的金属氧化物吸附剂、碳材料吸附剂、硅基介孔材料吸附剂的研究现状,吸附芳S 的羽络合作用、!一!相互作用和酸中心作用机理,以及固定床、移动床和模拟移动床的吸附分离工艺。
并指出开发新型高选择性、易回收芳s的吸附剂及吸附工艺是脱除柴油中芳s未来的发展趋势。
关键词:柴油;脱芳S;吸附剂;吸附机理;吸附分离工艺中图分类号:TE624文献标志码:A我国生产催化裂化(FCC)柴油的原油以重质油、劣质油主,其中的芳s体积分数达60%~ 80%。
芳S对柴油较,柴油中芳S 分数每降低10%,加3.0~3.5[1]o柴油中的芳s,仅可提高油,芳s原料,“三烯”,有要。
目,吸附分离油中芳s 的主要有催化加氢、剂、吸附分离。
催化加氢可以芳s[2-5],工艺用加氢,加氢理FCC油,工艺,操作,油。
芋剂抽提能有催化油中的芳s,溶剂较,需要经过多洁油,溶剂使用比例较高,工艺较,与加氢技术比无明显经济优。
吸附分离:近年来研究较多的脱除油中非理想组分的一种精制,使用仪器,操作缓和,对分离系的理化小,且吸附剂种类繁多,价格低廉,如活炭、分子筛、胶、氧化铝、硅藻土、白土[6-8],具有广阔的发展空间及应用前景。
工作绍了吸附工艺常用的吸附剂,并芳s的吸附机理和吸附工艺了综述。
1脱芳?吸附剂FCC柴油M分中富含芳s组分,主要为单环芳s、双环芳s以及稠环芳s,中50%以上是双环芳s包T、联和V类。
含水率和冻融循环对筋土界面剪切特性的影响
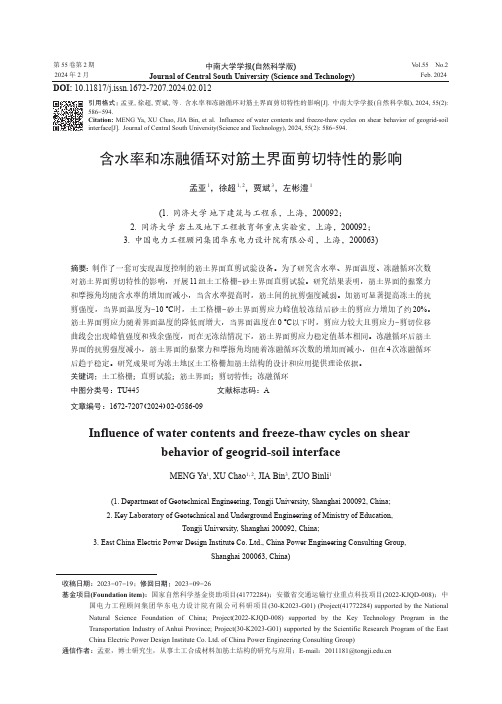
第 55 卷第 2 期2024 年 2 月中南大学学报(自然科学版)Journal of Central South University (Science and Technology)V ol.55 No.2Feb. 2024含水率和冻融循环对筋土界面剪切特性的影响孟亚1,徐超1, 2,贾斌3,左彬澧1(1. 同济大学 地下建筑与工程系,上海,200092;2. 同济大学 岩土及地下工程教育部重点实验室,上海,200092;3. 中国电力工程顾问集团华东电力设计院有限公司,上海,200063)摘要:制作了一套可实现温度控制的筋土界面直剪试验设备。
为了研究含水率、界面温度、冻融循环次数对筋土界面剪切特性的影响,开展11组土工格栅−砂土界面直剪试验。
研究结果表明,筋土界面的黏聚力和摩擦角均随含水率的增加而减小,当含水率提高时,筋土间的抗剪强度减弱。
加筋可显著提高冻土的抗剪强度,当界面温度为−10 ℃时,土工格栅−砂土界面剪应力峰值较冻结后砂土的剪应力增加了约20%。
筋土界面剪应力随着界面温度的降低而增大,当界面温度在0 ℃以下时,剪应力较大且剪应力−剪切位移曲线会出现峰值强度和残余强度,而在无冻结情况下,筋土界面剪应力稳定值基本相同。
冻融循环后筋土界面的抗剪强度减小,筋土界面的黏聚力和摩擦角均随着冻融循环次数的增加而减小,但在4次冻融循环后趋于稳定。
研究成果可为冻土地区土工格栅加筋土结构的设计和应用提供理论依据。
关键词:土工格栅;直剪试验;筋土界面;剪切特性;冻融循环中图分类号:TU445 文献标志码:A 文章编号:1672-7207(2024)02-0586-09Influence of water contents and freeze-thaw cycles on shearbehavior of geogrid-soil interfaceMENG Ya 1, XU Chao 1, 2, JIA Bin 3, ZUO Binli 1(1. Department of Geotechnical Engineering, Tongji University, Shanghai 200092, China;2. Key Laboratory of Geotechnical and Underground Engineering of Ministry of Education,Tongji University, Shanghai 200092, China;3. East China Electric Power Design Institute Co. Ltd., China Power Engineering Consulting Group,Shanghai 200063, China)收稿日期: 2023 −07 −19; 修回日期: 2023 −09 −26基金项目(Foundation item):国家自然科学基金资助项目(41772284);安徽省交通运输行业重点科技项目(2022-KJQD-008);中国电力工程顾问集团华东电力设计院有限公司科研项目(30-K2023-G01) (Project(41772284) supported by the National Natural Science Foundation of China; Project(2022-KJQD-008) supported by the Key Technology Program in the Transportation Industry of Anhui Province; Project(30-K2023-G01) supported by the Scientific Research Program of the East China Electric Power Design Institute Co. Ltd. of China Power Engineering Consulting Group)通信作者:孟亚,博士研究生,从事土工合成材料加筋土结构的研究与应用;E-mail :******************.cnDOI: 10.11817/j.issn.1672-7207.2024.02.012引用格式: 孟亚, 徐超, 贾斌, 等. 含水率和冻融循环对筋土界面剪切特性的影响[J]. 中南大学学报(自然科学版), 2024, 55(2): 586−594.Citation: MENG Ya, XU Chao, JIA Bin, et al. Influence of water contents and freeze-thaw cycles on shear behavior of geogrid-soil interface[J]. Journal of Central South University(Science and Technology), 2024, 55(2): 586−594.第 2 期孟亚,等:含水率和冻融循环对筋土界面剪切特性的影响Abstract:A set of temperature-controlled direct shear test apparatus for the interface of reinforced soil was developed. Eleven groups of direct shear tests were conducted to study the influence of water contents, interface temperatures, and freeze-thaw cycles on the shear behavior of the geogrid-soil interface. The results show that the cohesion and friction angle of the geogrid-soil interface both decrease with the increase of water contents, as does the shear strength at the geogrid-soil interface. The reinforcement can obviously improve the shear strength of the frozen soil. When the interface temperature is −10 ℃, the peak shear stress at the geogrid-soil interface increases by about 20% relative to the unreinforced soil. The reinforcement provided by the geogrid increaes as the interface temperature drops. The shear stress is higher and the shear stress-shear displacement will show the peak strength and residual strength when the interface temperature drops below 0 ℃, whereas in a non-frozen state, the interface exhibits lower shear stress with a consistent stable value. The shear strength of the geogrid-soil interface decreases after freeze-thawing. Furthermore, both the cohesion and friction angle of the geogrid-soil interface decrease with the increase of freeze-thaw cycles number but tend to stabilize after four freeze-thaw cycles. The research results have reference for the design and application of geogrid reinforced soil structures in permafrost and seasonal frozen regions.Key words: geogrid; direct shear test; geogrid-soil interface; shear behavior; freeze-thaw cycle加筋土结构具有抗震性能好、施工快、减碳环保等特点,被广泛应用于公路、铁路等工程中[1−3]。
各类基金项目名称英译
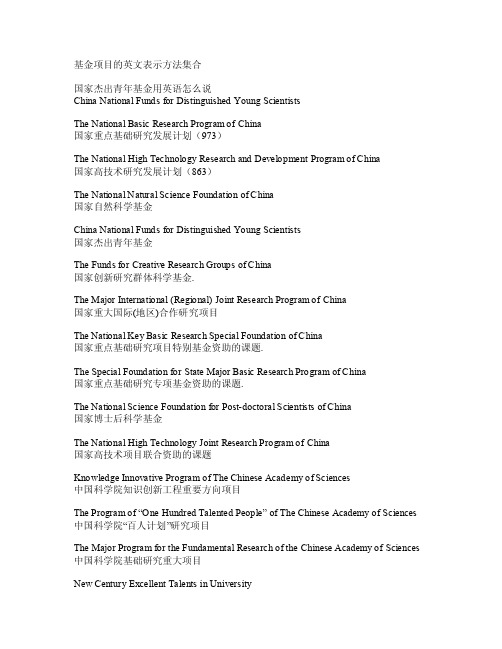
基金项目的英文表示方法集合国家杰出青年基金用英语怎么说C hinaNatio nal F undsfor D istin guish ed Yo ung S cient istsTheNatio nal B asicResea rch P rogra m ofChina国家重点基础研究发展计划(973)TheNatio nal H igh T echno logyResea rch a nd De velop mentProgr am of Chin a国家高技术研究发展计划(863)The N ation al Na tural Scie nce F ounda tionof Ch ina 国家自然科学基金China Nati onalFunds forDisti nguis hed Y oungScien tists国家杰出青年基金TheFunds forCreat ive R esear ch Gr oupsof Ch ina国家创新研究群体科学基金.T he Ma jor I ntern ation al (R egion al) J ointResea rch P rogra m ofChina国家重大国际(地区)合作研究项目T he Na tiona l Key Basi c Res earch Spec ial F ounda tionof Ch ina国家重点基础研究项目特别基金资助的课题.The Spec ial F ounda tionfor S tateMajor Basi c Res earch Prog ram o f Chi na国家重点基础研究专项基金资助的课题.Th e Nat ional Scie nce F ounda tionfor P ost-d octor al Sc ienti sts o f Chi na国家博士后科学基金T he Na tiona l Hig h Tec hnolo gy Jo int R esear ch Pr ogram of C hina国家高技术项目联合资助的课题Know ledge Inno vativ e Pro gramof Th e Chi neseAcade my of Scie nces中国科学院知识创新工程重要方向项目T he Pr ogram of “One H undre d Tal ented Peop le” o f The Chin ese A cadem y ofScien ces 中国科学院“百人计划”研究项目TheMajor Prog ram f or th e Fun damen tal R esear ch of theChine se Ac ademy of S cienc es 中国科学院基础研究重大项目Ne w Cen turyExcel lentTalen ts in Univ ersit y教育部新世纪优秀人才支持计划T he Im porta nt Pr oject of M inist ry of Educ ation教育部科学技术研究重大项目TheCheun g Kon g Sch olars Prog ramme教育部长江学者奖励计划T he Sc ienti fic R esear ch Fo undat ion o f the Stat e Hum an Re sourc e Min istry andthe E ducat ion M inist ry fo r Ret urned Chin ese S chola rs, C hina教育部和国家人事部留学回国人员基金T he Fo undat ion o f the Mini stryof Ed ucati on of Chin a for Outs tandi ng Yo ung T eache rs in Univ ersit y.教育部高等学校优秀青年教师研究基金The Foun datio n ofthe M inist ry of Educ ation of C hinafor R eturn ed Sc holar s教育部归国学者基金Th e Res earch Foun datio n fro m Min istry of E ducat ion o f Chi na教育部重大项目基金T he Tr ans-C entur y Tra ining Prog ram F ounda tionfor T alent s fro m the Mini stryofEd ucati on of Chin a教育部跨世纪人才训练基金TheScien ce Fo undat ion f or Po st Do ctora te Re searc h fro m the Mini stryof Sc ience andTechn ology of C hina科技部博士后基金Speci al Pr ophas e Pro jecton Ba sic R esear ch of TheNatio nal D epart mentof Sc ience andTechn ology科技部基础研究重大项目前期研究专项Grant forKey R esear ch It ems N o.2 i n “Cl imbin g” Pr ogram from theMinis try o f Sci enceand T echno logyof Ch ina 科技部攀登计划二号重点项目基金Spe ciali zed R esear ch Fu nd fo r the Doct oralProgr am of High er Ed ucati on高等学校博士学科点专项科研基金The S hangh ai “P hosph or” S cienc e Fou ndati on,Ch ina上海科技启明星基金资助The“Daw n”Pro gramof Sh angha i Edu catio n Com missi on上海市“曙光”计划The S hangh ai Po stdoc toral Sust entat ion F und上海市博士后基金M inist ry of Majo r Sci ence& Tec hnolo gy of Shan ghai上海市重大科技公关项目Th e Spe cialFound ation forYoung Scie ntist s ofZheji ang P rovin ce浙江省青年人才基金B eijin g Mun icipa l Sci enceand T echno logyProje ct北京市重大科技专项H eilon gjian g Pos tdoct oralGrant黑龙江省博士后资助基金G uangd ong N atura l Sci enceFound ation广东省自然科学基金项目T he "T enthfive" Obli gator y Bud get o f PLA军队“十五”指令性课题T he Fo k Yin g-Ton g Edu catio n Fou ndati on, C hina霍英东教育基金黑龙江省自然科学基金资助Su pport ed by Natu ral S cienc e Fou ndati on of Heil ongji ang P rovin ce of Chin a湖北省教育厅重点项目资助Supp orted by E ducat ional Comm issio n ofHubei Prov inceof Ch ina河南省杰出青年基金(9911)资助Su pport ed by Exce llent Yout h Fou ndati on of He’n an Sc ienti fic C ommit tee(项目编号:)河南省教育厅基金资助S uppor ted b y Fou ndati on of He’n an Ed ucati onalCommi ttee山西省青年科学基金(项目编号:)资助Supp orted by S hanxi Prov inceScien ce Fo undat ion f or Yo uths(项目编号:)山西省归国人员基金资助Supp orted by S hanxi Prov inceFound ation forRetur ness北京市自然科学基金资助Su pport ed by Beij ing M unici pal N atura l Sci enceFound ation上海市科技启明星计划(项目编号:)资助Su pport ed by Shan ghaiScien ce an d Tec hnolo gy De velop mentFunds(项目编号:)华北电力大学青年科研基金资助Suppo rtedby Yo uth F ounda tionof No rth-C hinaElect ric P owerUnive rsity华中师范大学自然科学基金资助Sup porte d byNatur al Sc ience Foun datio n ofCentr al Ch ina N ormal Univ ersit y东南大学基金(项目编号:)资助Suppo rtedby Fo undat ion o f Sou theas t ofUnive rsity(项目编号:)西南交通大学基础学科研究基金(项目编号:)资助Suppo rtedby Fo undat ion S cienc es So uthwe st Ji aoton g Uni versi ty(项目编号:)***科学技术厅科学家交流项目(项目编号:)Supp orted by J apanSTA S cient ist E xchan ge Pr ogram(项目编号:中国科学院基金资助Suppo rtedby Sc ience Foun datio n ofThe C hines e Aca demyof Sc ience s中国科学院九五重大项目(项目编号:)资助Suppo rtedby Ma jor S ubjec t ofThe C hines e Aca demyof Sc ience s(项目编号:)中国科学院院长基金特别资助Suppo rtedby Sp ecial Foun datio n ofPresi dentof Th e Chi neseAcade my of Scie nces中国科学院国际合作局重点项目资助Su pport ed by Bure au of Inte rnati onalCoope ratio n, Th e Chi neseAcade my of Scie nces中国科学院百人计划经费资助Suppo rtedby 100 Tal entsProgr ammeof Th e Chi neseAcade my of Scie ncesSuppo rtedby On e Hun dredPerso n Pro jectof Th e Chi neseAcade my of Scie nces中国科学院知识创新工程重大项目资助S uppor ted b y Kno wledg e Inn ovati on Pr oject of T he Ch inese Acad emy o f Sci ences Supp orted by K nowle dge I nnova tionProgr am of TheChine se Ac ademy of S cienc es 中国科学院西部之光基金(项目编号:)资助Supp orted by W est L ightFound ation of T he Ch inese Acad emy o f Sci ences(项目编号:)北京正负电子对撞机国家实验室重点课题资助Supp orted by B EPC N ation al La borat ory兰州重离子加速器国家实验室原子核理论中心基金资助Suppo rtedby Ce nterof Th eoret icalNucle ar Ph ysics, Nat ional Labo rator y ofHeavy IonAccel erato r ofLanzh ou国家自然科学基金(项目编号:)资助Su pport ed by Nati onalNatur al Sc ience Foun datio n ofChina(项目编号:)[Suppo rtedby NS FC(项目编号:)]国家自然科学基金重大项目资助Su pport ed by Majo r Pro gramof Na tiona l Nat uralScien ce Fo undat ion o f Chi na (1991483) 国家自然科学基金国际合作与交流项目(项目编号:)资助Sup porte d byProje cts o f Int ernat ional Coop erati on an d Exc hange s NSF C(项目编号:)国家重点基础研究发展规划项目(项目编号:)资助 (973计划项目)Sup porte d byMajor Stat e Bas ic Re searc h Dev elopm ent P rogra m(项目编号:)Suppo rtedby Ch ina M inist ry of Scie nce a nd Te chnol ogy u nderContr act(项目编号:)Sup porte d byState KeyDevel opmen t Pro gramof (f or) B asicResea rch o f Chi na(项目编号:)国家高技术研究发展计划(863计划)资助Su pport ed by Nati onalHighTechn ology Rese archand D evelo pment Prog ram o f Chi na国家重大科学工程二期工程基金资助Supp orted by N ation al Im porta nt Pr oject on S cienc e-Pha se Ⅱof NS RL国家攀登计划—B课题资助Sup porte d byNatio nal C limb—B Pla n国家杰出青年科学基金资助Supp orted by N ation al Na tural Scie nce F undsfor D istin guish ed Yo ung S chola r国家科技部基金资助Su pport ed by Stat e Com missi on of Scie nce T echno logyof Ch ina(科委)Su pport ed by Mini stryof Sc ience andTechn ology of C hina中国博士后科学基金Supp orted by C hinaPostd octor al Sc ience Foun datio n海峡两岸自然科学基金(项目编号:)共同资助Supp orted by S cienc e Fou ndati on of Twosides of S trait(项目编号:)核工业科学基金资助Suppo rtedby Sc ience Foun datio n ofChine se Nu clear Indu stry国家教育部科学基金资助Su pport ed by Scie nce F ounda tionof Th e Chi neseEduca tionCommi ssion (教委)Supp orted by S cienc e Fou ndati on of Mini stryof Ed ucati on of Chin a国家教育部博士点专项基金资助Su pport ed by Doct oralFundof Mi nistr y ofEduca tionof Ch ina国家教育部回国人员科研启动基金资助Su pport ed by Scie ntifi c Res earch Foun datio n for Retu rnedSchol ars,Minis try o fEdu catio n ofChina国家教育部优秀青年教师基金资助Su pport ed by Scie nce F ounda tionfor T he Ex celle nt Yo uth S chola rs of Mini stryofEd ucati on of Chin a高等学校博士学科点专项科研基金资助Supp orted by R esear ch Fu nd fo r the Doct oralProgr am of High er Ed ucati on of Chin aSup porte d byDocto ral P rogra m Fou ndati on of Inst ituti ons o f Hig her E ducat ion o f Chi na 国家自然科学基金中文标注:国家自然科学基金资助项目批准号********英标标注:Proj ect ******** (项目批准号)suppo rtedby Na tiona l Nat uralScien ceFo undat ion o f Chi na,可缩写为:Pr oject ********* supp orted by N SFC2、浙江省自然科学基金中文标注:浙江省自然科学基金资助项目英文标注:The Proj ect S uppor ted b y Zhe jiang Prov incia l Nat uralScien ce Fo undat ion o f Chi na3、教育部高等学校博士学科点专科研基金中文标注:高等学校博士学科点专项科研基金资助课题英文标注:The Rese archFundfor t he De ctora l Pro gramof Hi gherEduca tion可缩写为:R FDP4、教育部高等学校骨干教师资助计划中文标注:高等学校骨干教师资助计划资助英文标注:Supp orted by F ounda tionfor U niver sityKey T eache r bythe M inist ry of Educ ation5、教育部霍英东教育基金项目中文标注:教育部霍英东教育基金资助6、教育部留学回国人员科研启动基金中文标注:教育部留学回国人员科研启动基金资助英文标注:The Proj ect S ponso red b y the Scie ntifi c Res earch Foun datio n for theRetur ned O verse as Ch inese Scho lars, Stat e Edu catio n Min istry可缩写为::TheProje ct sp onsor ed by SRFfor R OCS,SEM)7、教育部优秀青年教师资助计划项目中文标注:教育部优秀青年教师资助计划项目英文标注:Su pport ed by theExcel lentYoung Teac hersPorgr am of MOE, P.R.C.可缩写为EYTP8、教育部跨世纪优秀人才培养计划中文标注:跨世纪优秀人才培养计划英文标注:T rans-Centu ry Tr ainin g Pro gramm e Fou ndati on fo r the Tale nts b y theMini stryof Ed ucati on9、教育部新世纪优秀人才支持计划中文标注:新世纪优秀人才支持计划资助英文标注:Su pport ed by Prog ram f or Ne w Cen turyExcel lentTalen ts in Univ ersit y(英文缩写“NCE T”)10、教育部长江学者与创新团队发展计划中文标注:长江学者和创新团队发展计划资助英文标注:Su pport ed by Prog ram f or Ch angji ang S chola rs an d Inn ovati ve Re searc hTea m inUnive rsity(缩写为“PCSIR T”)基金项目英文翻译及基金资助书写格式基金项目英文翻译1 国家高技术研究发展计划资助项目(863计划)(No.)Thi s wor k was supp orted by a gran t fro m the Nati onalHighTechn ology Rese archand D evelo pment Prog ram o f Chi na (863 Pr ogram) (No. )2国家自然科学基金资助项目(N o. )Gener al Pr ogram(面上项目), Ke y Pro gram(重点项目), Maj or Pr ogram(重大项目)Thi s wor k was supp orted by a gran t fro m the Nati onalNatur al Sc ience Foun datio n ofChina(No.)3国家“九五”攻关项目(No.)Thi s wor k was supp orted by a gran t fro m the Nati onalKey T echno logie s R & D Pr ogram of C hinadurin g the 9thFive-YearPlanPerio d (No. )4中国科学院“九五”重大项目(No. )This work wassuppo rtedby agrant from theMajor Prog ramsof th e Chi neseAcade my of Scie ncesdurin g the 9thFive-YearPlanPerio d (No. )5中国科学院重点资助项目(No. )T his w ork w as su pport ed by a gr ant f rom t he Ke y Pro grams of t he Ch inese Acad emy o f Sci ences (No. )6“九五”国家医学科技攻关基金资助项目(N o. )Thisworkwas s uppor ted b y a g rantfromthe N ation al Me dical Scie nce a nd Te chniq ue Fo undat ion d uring the9th F ive-Y ear P lan P eriod(No.)7江苏省科委应用基础基金资助项目 (No. )Th is wo rk wa s sup porte d bya gra nt fr om th e App liedBasic Rese archProgr ams o fSc ience andTechn ology Comm issio n Fou ndati on of Jian gsu P rovin ce (N o. )8 国家教育部博士点基金资助项目(No. )Th is wo rk wa s sup porte d bya gra nt fr om th e Ph.D. Pr ogram s Fou ndati on of Mini stryof Ed ucati on of Chin a (No. )9中国科学院上海分院择优资助项目(No. )Th is wo rk wa s sup porte d bya gra nt fr om Ad vance d Pro grams of S hangh ai Br anch, theChine se Ac ademy of S cienc es (N o. )10 国家重点基础研究发展规划项目(973计划)(No. )This work wassuppo rtedby agrant from theMajor Stat e Bas ic Re searc hDev elopm ent P rogra m ofChina (973 Prog ram)(No.)11国家杰出青年科学基金(No.)Thi s wor k was supp orted by a gran t fro m Nat ional Scie nce F und f or Di sting uishe dYou ng Sc holar s (No. )12 海外香港青年学者合作研究基金(No. )Th is wo rk wa s sup porte d bya gra nt fr om Jo int R esear ch Fu nd fo r You ng Sc holar s inHongKongand A broad(No.)中国科学院基金资助S uppor ted b y Sci enceFound ation of T he Ch inese Acad emy o f Sci ences中国科学院九五重大项目(项目编号:)资助Suppo rtedby Ma jor S ubjec t ofThe C hines e Aca demyof Sc ience s(项目编号:)中国科学院院长基金特别资助Supp orted by S pecia l Fou ndati on of Pres ident of T he Ch inese Acad emy o f Sci ences中国科学院国际合作局重点项目资助Su pport ed by Bure au of Inte rnati onalCoope ratio n, Th e Chi neseAcade my of Scie nces中国科学院百人计划经费资助S uppor ted b y 100 Tale nts P rogra m ofThe C hines e Aca demyof Sc ience sSup porte d byOne H undre d Per son P rojec t ofThe C hines e Aca demyof Sc ience s中国科学院知识创新工程重大项目资助Suppo rtedby Kn owled ge In novat ion P rojec t ofThe C hines e Aca demyof Sc ience sSup porte d byKnowl edgeInnov ation Prog ram o f The Chin ese A cadem y ofScien ces中国科学院西部之光基金(项目编号:)资助Su pport ed by West Ligh t Fou ndati on of TheChine se Ac ademy of S cienc es(项目编号:)北京正负电子对撞机国家实验室重点课题资助S uppor ted b y BEP C Nat ional Labo rator y兰州重离子加速器国家实验室原子核理论中心基金资助Sup porte d byCente r ofTheor etica l Nuc learPhysi cs, N ation al La borat ory o f Hea vy Io n Acc elera tor o f Lan zhou国家自然科学基金(项目编号:)资助Supp orted by N ation al Na tural Scie nce F ounda tionof Ch ina(项目编号:)[Suppo rtedby NS FC(项目编号:)]国家自然科学基金重大项目资助S uppor ted b y Maj or Pr ogram of N ation al Na tural Scie nce F ounda tionof Ch ina (1991483) 国家自然科学基金国际合作与交流项目(项目编号:)资助S uppor ted b y Pro jects of I ntern ation al Co opera tionand E xchan ges N SFC(项目编号:)国家重点基础研究发展规划项目(项目编号:)资助 (973计划项目)Supp orted by M ajorState Basi c Res earch Deve lopme nt Pr ogram(项目编号:)Supp orted by C hinaMinis try o f Sci enceand T echno logyunder Cont ract(项目编号:)Suppo rtedby St ate K ey De velop mentProgr am of (for) Bas ic Re searc h ofChina(项目编号:)国家高技术研究发展计划(863计划)资助Sup porte d byNatio nal H igh T echno logyResea rch a nd De velop mentProgr am of Chin a 国家重大科学工程二期工程基金资助S uppor ted b y Nat ional Impo rtant Proj ect o n Sci ence-PhaseⅡ of NSRL国家攀登计划—B课题资助S uppor ted b y Nat ional Clim b—B P lan国家杰出青年科学基金资助Sup porte d byNatio nal N atura l Sci enceFunds forDisti nguis hed Y oungSchol ar国家科技部基金资助Su pport ed by Stat e Com missi on of Scie nce T echno logyof Ch ina(科委)Su pport ed by Mini stryof Sc ience andTechn ology of C hina中国博士后科学基金Suppo rtedby Ch ina P ostdo ctora l Sci enceFound ation海峡两岸自然科学基金(项目编号:)共同资助Supp orted by S cienc e Fou ndati on of Twosides of S trait(项目编号:)核工业科学基金资助Supp orted by S cienc e Fou ndati on of Chin ese N uclea r Ind ustry国家教育部科学基金资助Su pport ed by Scie nce F ounda tionof Th e Chi neseEduca tionCommi ssion (教委)Supp orted by S cienc e Fou ndati on of Mini stryof Ed ucati on of Chin a国家教育部博士点专项基金资助Sup porte d byDocto ral F und o f Min istry of E ducat ion o f Chi na国家教育部回国人员科研启动基金资助Supp orted by S cient ificResea rch F ounda tionfor R eturn ed Sc holar s, Mi nistr y ofEduca tionof Ch ina国家教育部优秀青年教师基金资助Suppo rtedby Sc ience Foun datio n for TheExcel lentYouth Scho larsof Mi nistr y ofEduca tionof Ch ina高等学校博士学科点专项科研基金资助Sup porte d byResea rch F und f or th e Doc toral Prog ram o f Hig her E ducat ion o f Chi naSu pport ed by Doct oralProgr am Fo undat ion o f Ins titut ionsof Hi gherEduca tionof Ch ina 霍英东教育基金会青年教师基金资助黑龙江省自然科学基金资助S uppor ted b y Nat uralScien ce Fo undat ion o f Hei longj iangProvi nce o f Chi na湖北省教育厅重点项目资助Supp orted by E ducat ional Comm issio n ofHubei Prov inceof Ch ina河南省杰出青年基金(9911)资助Sup porte d byExcel lentYouth Foun datio n ofHe’na n Sci entif ic Co mmitt ee(项目编号:)河南省教育厅基金资助S uppor ted b y Fou ndati on of He’n an Ed ucati onalCommi ttee山西省青年科学基金(项目编号:)资助Sup porte d byShanx i Pro vince Scie nce F ounda tionfor Y ouths(项目编号:)山西省归国人员基金资助Su pport ed by Shan xi Pr ovinc e Fou ndati on fo r Ret urnes s北京市自然科学基金资助S uppor ted b y Bei jingMunic ipalNatur al Sc ience Foun datio n上海市科技启明星计划(项目编号:)资助Suppo rtedby Sh angha i Sci enceand T echno logyDevel opmen t Fun ds(项目编号:)华北电力大学青年科研基金资助Su pport ed by Yout h Fou ndati on of Nort h-Chi na El ectri c Pow er Un ivers ity华中师范大学自然科学基金资助S uppor ted b y Nat uralScien ce Fo undat ion o f Cen tralChina Norm al Un ivers ity东南大学基金(项目编号:)资助Su pport ed by Foun datio n ofSouth eastof Un ivers ity(项目编号:)西南交通大学基础学科研究基金(项目编号:)资助Supp orted by F ounda tionScien ces S outhw est J iaoto ng Un ivers ity(项目编号:)日本科学技术厅科学家交流项目(项目编号:)S uppor ted b y Jap an ST A Sci entis t Exc hange Prog ram (项目编号:)Part 1:国家自然科学基金(项目编号:)资助S uppor ted b y Nat ional Natu ral S cienc e Fou ndati on of Chin a(项目编号:)[Supp orted by N SFC(项目编号:)]国家自然科学基金重大项目资助S uppor ted b y Maj or Pr ogram of N ation al Na tural Scie nce F ounda tionof Ch ina (1991483) 国家自然科学基金国际合作与交流项目(项目编号:)资助Su pport ed by Proj ectsof In terna tiona l Coo perat ion a nd Ex chang es NS FC(项目编号:)国家重点基础研究发展规划项目(项目编号:)资助 (973计划项目)Sup porte d byMajor Stat e Bas ic Re searc h Dev elopm ent P rogra m(项目编号:)Suppo rtedby Ch ina M inist ry of Scie nce a nd Te chnol ogy u nderContr act(项目编号:)Sup porte d byState KeyDevel opmen t Pro gramof (f or) B asicResea rch o f Chi na(项目编号:)国家高技术研究发展计划(863计划)资助Su pport ed by Nati onalHighTechn ology Rese archand D evelo pment Prog ram o f Chi na国家重大科学工程二期工程基金资助Supp orted by N ation al Im porta nt Pr oject on S cienc e-Pha se Ⅱof NS RL国家攀登计划—B课题资助Sup porte d byNatio nal C limb—B Pla n国家杰出青年科学基金资助Supp orted by N ation al Na tural Scie nce F undsfor D istin guish ed Yo ung S chola r国家科技部基金资助Su pport ed by Stat e Com missi on of Scie nce T echno logyof Ch ina(科委)Su pport ed by Mini stryof Sc ience andTechn ology of C hina中国科学院基金资助Sup porte d byScien ce Fo undat ion o f The Chin ese A cadem y ofScien ces中国科学院九五重大项目(项目编号:)资助Sup porte d byMajor Subj ect o f The Chin ese A cadem y ofScien ces(项目编号:)中国科学院院长基金特别资助Sup porte d bySpeci al Fo undat ion o f Pre siden t ofThe C hines e Aca demyof Sc ience s中国科学院国际合作局重点项目资助Suppo rtedby Bu reauof In terna tiona l Coo perat ion,The C hines e Aca demyof Sc ience s 中国科学院百人计划经费资助Sup porte d by100 T alent s Pro gramm e ofThe C hines e Aca demyof Sc ience sSup porte d byOne H undre d Per son P rojec t ofThe C hines e Aca demyof Sc ience s中国科学院知识创新工程重大项目资助Supp orted by K nowle dge I nnova tionProje ct of TheChine se Ac ademy of S cienc esSu pport ed by Know ledge Inno vatio n Pro gramof Th e Chi neseAcade my of Scie nces中国科学院西部之光基金(项目编号:)资助Su pport ed by West Ligh t Fou ndati on of TheChine se Ac ademy of S cienc es(项目编号:)北京正负电子对撞机国家实验室重点课题资助Su pport ed by BEPC Nati onalLabor atory兰州重离子加速器国家实验室原子核理论中心基金资助Sup porte d byCente r ofTheor etica l Nuc learPhysi cs, N ation al La borat ory o f Hea vy Io n Acc elera tor o f Lan zhou中国博士后科学基金Supp orted by C hinaPostd octor al Sc ience Foun datio n海峡两岸自然科学基金(项目编号:)共同资助Supp orted by S cienc e Fou ndati on of Twosides of S trait(项目编号:)核工业科学基金资助Suppo rtedby Sc ience Foun datio n ofChine se Nu clear Indu stry国家教育部科学基金资助Su pport ed by Scie nce F ounda tionof Th e Chi neseEduca tionCommi ssion (教委)Supp orted by S cienc e Fou ndati on of Mini stryof Ed ucati on of Chin a国家教育部博士点专项基金资助Su pport ed by Doct oralFundof Mi nistr y ofEduca tionof Ch ina国家教育部回国人员科研启动基金资助Su pport ed by Scie ntifi c Res earch Foun datio n for Retu rnedSchol ars,Minis try o fEdu catio n ofChina国家教育部优秀青年教师基金资助Su pport ed by Scie nce F ounda tionfor T he Ex celle nt Yo uth S chola rs of Mini stryofEd ucati on of Chin a高等学校博士学科点专项科研基金资助Supp orted by S pecia lized Rese archFundfor t he Do ctora l Pro gramof Hi gherEduca tion霍英东教育基金会青年教师基金资助Su pport ed by theFok Y ing-T ong E ducat ion F ounda tion, Chin a (Gr ant N o. )黑龙江省自然科学基金资助Suppo rtedby Na tural Scie nce F ounda tionof He ilong jiang Prov inceof Ch ina湖北省教育厅重点项目资助Su pport ed by Educ ation al Co mmiss ion o f Hub ei Pr ovinc e ofChina河南省杰出青年基金(9911)资助Suppo rtedby Ex celle nt Yo uth F ounda tionof He’nanScien tific Comm ittee(项目编号:)河南省教育厅基金资助Supp orted by F ounda tionof He’nanEduca tiona l Com mitte e山西省青年科学基金(项目编号:)资助Su pport ed by Shan xi Pr ovinc e Sci enceFound ation forYouth s(项目编号:)山西省归国人员基金资助Su pport ed by Shan xi Pr ovinc e Fou ndati on fo r Ret urnes s北京市自然科学基金资助Suppo rtedby Be ijing Muni cipal Natu ral S cienc e Fou ndati on上海市科技启明星计划(项目编号:)资助Suppo rtedby Sh angha i Sci enceand T echno logyDevel opmen t Fun ds(项目编号:)华北电力大学青年科研基金资助Sup porte d byYouth Foun datio n ofNorth-Chin a Ele ctric Powe r Uni versi ty华中师范大学自然科学基金资助S uppor ted b y Nat uralScien ce Fo undat ion o f Cen tralChina Norm al Un ivers ity东南大学基金(项目编号:)资助Sup porte d byFound ation of S outhe ast o f Uni versi ty(项目编号:)西南交通大学基础学科研究基金(项目编号:)资助Sup porte d byFound ation Scie ncesSouth westJiaot ong U niver sity(项目编号:)日本科学技术厅科学家交流项目(项目编号:)Sup porte d byJapan STAScien tistExcha nge P rogra m (项目编号:)Par t 2:1、国家自然科学基金资助项目凡是国家自然科学基金资助项目的研究成果,必须严格按规定进行标注才算有效,否则基金委将不予承认。
青藏高原巨厚地壳:生长、加厚与演化
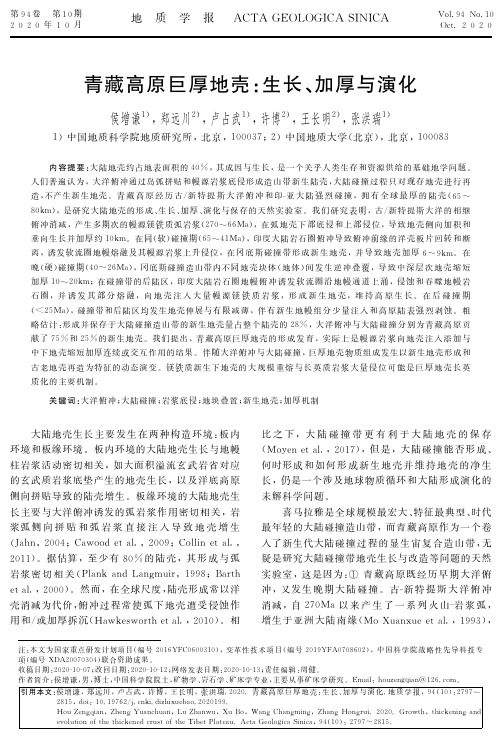
学研究予以准确标定,其物质演变 及其 深部过 程也
可被同位素填图和地球物理探测予以明确限定。
对 于 青 藏 高 原 巨 厚 地 壳 的 成 因 与 生 长 ,前 人 已
提出了若干 模 式,如 印 度 大 陆 地 壳 楔 入 模 式 (Zhao Wulingand Morgan,1985;Nábeleketal.,2009)、 地 壳 缩 短 与 加 厚 模 式 (Englandand Houseman, 1986)、新 生 幔 源 岩 浆 注 入 模 式 (Niu Yaolingetal., 2013;HouZengqianetal.,2015a)、地壳连 续生 长 加厚模式(ZhuDichengetal.,2017)等。 然 而,地 球物理探测发现,印度大陆岩石圈地幔可 能 以低 缓
注:本文为国家重点研发计划项目(编号 2016YFC0600310),变革 性 技 术 项 目(编 号 2019YFA0708602),中 国 科 学 院 战 略 性 先 导 科 技 专 项(编号 XDA20070304)联合资助成果。 收 稿 日 期 :20201007;改 回 日 期 :20201012;网 络 发 表 日 期 :20201013;责 任 编 辑 :周 健 。 作 者 简 介 :侯 增 谦 ,男 ,博 士 ,中 国 科 学 院 院 士 ,矿 物 学 、岩 石 学 、矿 床 学 专 业 ,主 要 从 事 矿 床 学 研 究 。Email:houzengqian@126.com。
本文拟 在 前 人 研 究 基 础 上,结 合 笔 者 研 究 成 果,从物质记录与构 造 变 形 两 个 角 度,系 统 剖 析 青 藏高原从 俯 冲 到 碰 撞 全 过 程 的 地 壳 生 长 与 加 厚 原 因,半定量评估 俯 冲 与 碰 撞、岩 浆 与 构 造 对 地 壳 生 长和加厚的相对贡献,深入揭 示大 陆碰 撞引 发地 壳 生长与物质演化的作用机制和深部过程。
Y. Geometric stability switch criteria in delay differential systems with delay dependent p
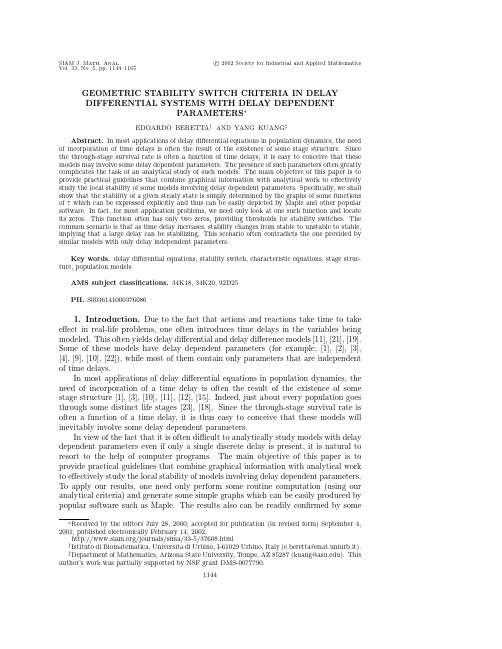
土壤微塑料影响植物生长的因素与机制研究进展

塑料作为一种被广泛应用的有机合成聚合物材料,在为我们生活提供便利的同时,也带来了后续的环境问题。
据估算,到2050年,将有大约12000万t 塑料垃圾被埋入垃圾填埋场或自然环境中[1],塑料垃圾进入到环境后会逐渐破碎变成微塑料(<5mm ),导致其在土壤和水体中的丰度逐年递增[2-3]。
2015年的第二届联合国环境大会已将微塑料污染列为环境与生态领域的第二大科学问题[3]。
目前,人们对水体中微塑料的认识已较为系统[4-6],土壤微塑料逐步成为新的研究热点[7-8]。
2012年Rillig [9]首次提出微塑料会影响土壤理化性质,这引起了人们对土壤微塑料的关注,后续研究表明微塑料可被植物吸收并积累[10-11],最终通过食物链进入人体。
厘清微塑料对植物生长的影响及其机制,有助于系统掌握其在土壤-植物体陈欣,郭薇,李济之,等.土壤微塑料影响植物生长的因素与机制研究进展[J].农业环境科学学报,2024,43(3):488-495.CHEN X,GUO W,LI J Z,et al.Research progress on the influencing factors and mechanisms of soil microplastics on plant growth[J].Journal of Agro-Environment Science ,2024,43(3):488-495.土壤微塑料影响植物生长的因素与机制研究进展陈欣1,郭薇1,2,李济之1,2,迟光宇1*(1.中国科学院沈阳应用生态研究所,污染生态与环境工程重点实验室,沈阳110016;2.中国科学院大学,北京100049)Research progress on the influencing factors and mechanisms of soil microplastics on plant growthCHEN Xin 1,GUO Wei 1,2,LI Jizhi 1,2,CHI Guangyu 1*(1.Key Laboratory of Pollution Ecology and Environmental Engineering,Institute of Applied Ecology,Chinese Academy of Sciences,Shenyang 110016,China ;2.University of Chinese Academy of Sciences,Beijing 100049,China )Abstract :Microplastics in soil can affect plant growth in a variety of ways,accumulate in plants,and eventually enter the human body via the food chain.Clarifying the mechanisms and main factors whereby microplastics influence plant growth can contribute to a systematic understanding of their environmental behavior in soil-plant systems.Both the occurrence state and physicochemical characteristics of microplastics can influence their effects on plants.In this paper,from the perspectives of particle size,shape,concentration,and type,plastic additives,and aging degree of microplastics,we review the main factors and mechanisms underlying the effects of soil microplastics on plant growth.The key direction of future research is proposed,which will provide a reference for further clarifying the impact of microplastics on soil ecosystems.Keywords :soil;microplastics;plant;influencing factor;mechanism of action收稿日期:2023-04-13录用日期:2023-06-19作者简介:陈欣(1968—),男,辽宁沈阳人,博士,研究员,研究方向为农业生态。
胶东-朝鲜半岛中生代金成矿作用
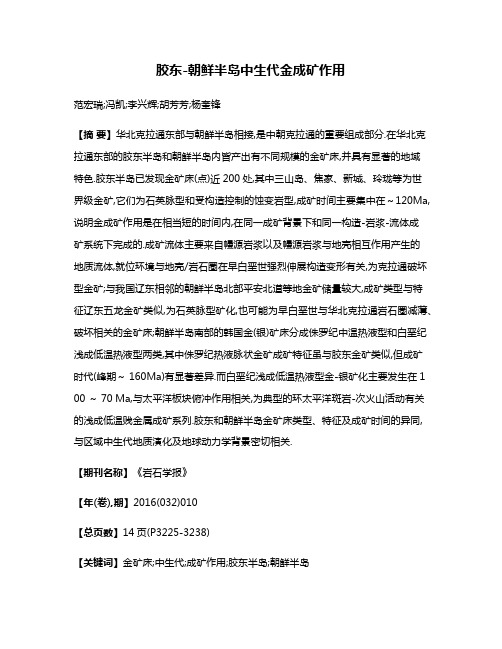
胶东-朝鲜半岛中生代金成矿作用范宏瑞;冯凯;李兴辉;胡芳芳;杨奎锋【摘要】华北克拉通东部与朝鲜半岛相接,是中朝克拉通的重要组成部分.在华北克拉通东部的胶东半岛和朝鲜半岛内皆产出有不同规模的金矿床,并具有显著的地域特色.胶东半岛已发现金矿床(点)近200处,其中三山岛、焦家、新城、玲珑等为世界级金矿,它们为石英脉型和受构造控制的蚀变岩型,成矿时间主要集中在~120Ma,说明金成矿作用是在相当短的时间内,在同一成矿背景下和同一构造-岩浆-流体成矿系统下完成的.成矿流体主要来自幔源岩浆以及幔源岩浆与地壳相互作用产生的地质流体,就位环境与地壳/岩石圈在早白垩世强烈伸展构造变形有关,为克拉通破坏型金矿;与我国辽东相邻的朝鲜半岛北部平安北道等地金矿储量较大,成矿类型与特征辽东五龙金矿类似,为石英脉型矿化,也可能为早白垩世与华北克拉通岩石圈减薄、破坏相关的金矿床;朝鲜半岛南部的韩国金(银)矿床分成侏罗纪中温热液型和白垩纪浅成低温热液型两类,其中侏罗纪热液脉状金矿成矿特征虽与胶东金矿类似,但成矿时代(峰期~ 160Ma)有显著差异.而白垩纪浅成低温热液型金-银矿化主要发生在1 00 ~ 70 Ma,与太平洋板块俯冲作用相关,为典型的环太平洋斑岩-次火山活动有关的浅成低温贱金属成矿系列.胶东和朝鲜半岛金矿床类型、特征及成矿时间的异同,与区域中生代地质演化及地球动力学背景密切相关.【期刊名称】《岩石学报》【年(卷),期】2016(032)010【总页数】14页(P3225-3238)【关键词】金矿床;中生代;成矿作用;胶东半岛;朝鲜半岛【作者】范宏瑞;冯凯;李兴辉;胡芳芳;杨奎锋【作者单位】中国科学院地质与地球物理研究所矿产资源研究重点实验室,北京100029;中国科学院大学,北京100049;中国科学院地质与地球物理研究所矿产资源研究重点实验室,北京100029;中国科学院大学,北京100049;中国科学院地质与地球物理研究所矿产资源研究重点实验室,北京100029;中国科学院大学,北京100049;中国科学院地质与地球物理研究所矿产资源研究重点实验室,北京100029;中国科学院大学,北京100049;中国科学院地质与地球物理研究所矿产资源研究重点实验室,北京100029;中国科学院大学,北京100049【正文语种】中文【中图分类】P618.51Fan HR, Feng K, Li XH, Hu FF and Yang KF. 2016. Mesozoic gold mineralization in the Jiaodong and Korean peninsulas. Acta Petrologica Sinica, 32(10):3225-3238位于华北克拉通东部的胶东半岛是我国最重要的金矿产地,以0.2%的国土面积占有了全国近四分之一的黄金储量,目前已查明金资源量和黄金产量均居全国之首。
小学上册第9次英语第5单元综合卷(有答案)
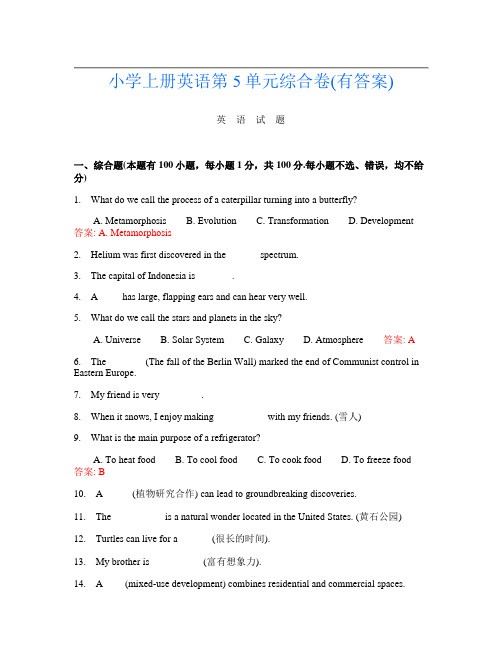
小学上册英语第5单元综合卷(有答案)英语试题一、综合题(本题有100小题,每小题1分,共100分.每小题不选、错误,均不给分)1.What do we call the process of a caterpillar turning into a butterfly?A. MetamorphosisB. EvolutionC. TransformationD. Development 答案: A. Metamorphosis2.Helium was first discovered in the ______ spectrum.3.The capital of Indonesia is _______.4. A ____ has large, flapping ears and can hear very well.5.What do we call the stars and planets in the sky?A. UniverseB. Solar SystemC. GalaxyD. Atmosphere答案: A6.The _______ (The fall of the Berlin Wall) marked the end of Communist control in Eastern Europe.7.My friend is very ________.8.When it snows, I enjoy making __________ with my friends. (雪人)9.What is the main purpose of a refrigerator?A. To heat foodB. To cool foodC. To cook foodD. To freeze food答案: B10. A _____ (植物研究合作) can lead to groundbreaking discoveries.11.The __________ is a natural wonder located in the United States. (黄石公园)12.Turtles can live for a ______ (很长的时间).13.My brother is __________ (富有想象力).14. A ____(mixed-use development) combines residential and commercial spaces.15.What is the name of the famous ancient ruins in Mexico?A. TeotihuacanB. Machu PicchuC. Angkor WatD. Petra答案: A16.We visit the ______ (自然史博物馆) to learn about fossils.17.The discovery of ________ changed the course of history.18. A dolphin leaps gracefully out of the _______ and splashes down again.19.I enjoy playing ________ with my family.20.I like to ___ (play/watch) games.21.What do we call a young female goat?A. KidB. CalfC. LambD. Foal答案:A.Kid22.My friend is __________ (聪明绝顶).23.The _______ can change its shape with the seasons.24.The _____ (养分) in the soil is vital for plant health.25.What is the term for a young goat?A. CalfB. KidC. LambD. Foal答案: B26.An electric motor converts electrical energy into _______ energy.27.Animals that have scales are typically __________.28.The capital of Bonaire is __________.29.My favorite animal is a ______ (dolphin).30. A __________ is a reaction that involves a change in temperature.31.The first successful cloning of a mammal was of _____.32.I like to go ________ (爬山) with my friends.33.The ______ (小鸟) builds a nest for its eggs.34.My _____ (仓鼠) runs on its wheel.35.The ______ helps us learn about communication.36.The painting is very ___ (colorful).37.I often visit my ____.38.I can see a ______ in the sky. (bird)39. A strong acid has a pH less than ______.40.The atomic number of an element tells you the number of _____ (protons) it has.41.What do we call the part of the brain that controls balance?A. CerebellumB. CerebrumC. BrainstemD. Cortex答案:A42.The __________ is a famous natural landmark in the United States. (黄石公园)43.The capital of Ecuador is __________.44.The iguana is often seen basking in the ______ (阳光).45.The __________ (农业) is important for our economy.46.The ______ (小龙) is a mythical creature often found in ______ (故事).47.What is the term for a baby capybara?A. PupB. KitC. CalfD. Hatchling答案:c48.The fish swims in the ___. (water)49.The chemical formula for calcium chloride is ______.50.The ancient Romans practiced ________ (宗教多元).51.I want to _____ (go/stay) at home.52.The speed of light is very ______.53.What do we call a baby dog?A. KittenB. PuppyC. CalfD. Chick答案:B54.The chemical formula for yttrium oxide is _____.55.The Earth's surface is shaped by both climatic and ______ factors.56.Understanding plant _____ (结构) helps in gardening.57.The _____ (spoon) is shiny.58.The _____ (温带雨林) hosts a variety of plant species.59.The balloon is ______ (floating) in the air.60.The river is ______ (calm) and clear.61. A solution with a pH of contains more ______ than a solution with a pH of .62. A ____ is a large animal that can be trained to work.ets are made of ice, dust, and ______.64.__________ are used in the beauty industry for skincare.65.The _____ is a phenomenon where the moon blocks the sun.66.My cat enjoys the warmth of the _______ (阳光).67.The __________ is important for keeping bones strong.68.The __________ is the area of land between two rivers.69.The __________ (历史的深度剖析) reveals nuances.70.Certain plants can ______ (提供) habitat for endangered species.71. A _______ can measure the amount of energy consumed by a device.72.The ________ was a significant treaty that fostered diplomatic relations.73.The chemical symbol for silver is ________.74.I like to draw pictures of my ________ (玩具名) and imagine their adventures.75.I share my toys with my ______. (我和我的______分享玩具。
石墨烯表面等离激元特性研究
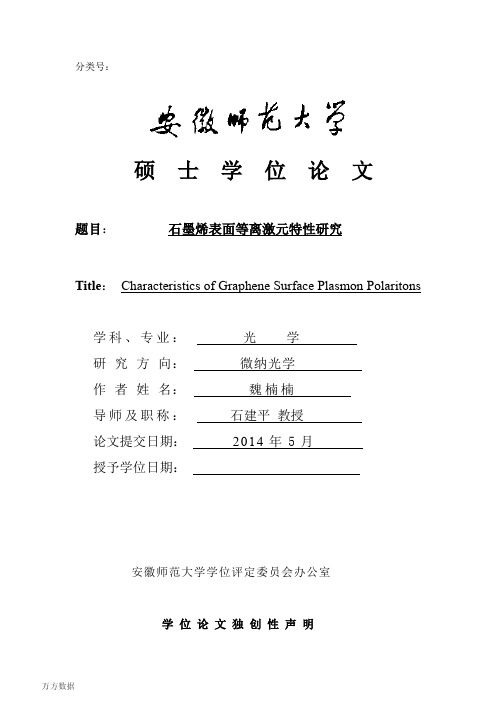
硕
题目:
士
学
位 论
文
石墨烯表面等离激元特性研究
Title: Characteristics of Graphene Surface Plasmon Polaritons
学科、专业: 研 究 方 向: 作 者 姓 名: 导师及职称: 论文提交日期: 授予学位日期:
光
学
微纳光学 魏楠楠 石建平 教授 2 0 14 年 5 月
结束语 ...................................................................................................................... 65 参考文献 ................................................................................................................. 67
1
万方数据
的模型,并对其透射特性进行分析;最后,详细分析石墨烯条带阵列 激发表面等离激元的透射特性,得出,石墨烯阵列周期宽度的变化可 以实现对表面等离子激元的调制。 关键字:表面等离激元 石墨烯 FDTD SPPs 调制
2
万方数据
Abstract
Surface plasmon polaritons(SPPs) ,which has some distinguishing features including abnormal transmission through small holes and enhanced greatly local electromagnetic field, provides a new technical approach for solving the conventional optical system (device) diffraction limit . SPPs that has a good application for super-resolution imaging, high resolution imaging lithography, a new micro-nano optoelectronic device design and other aspects, is currently a hot research in micro and nano optics. Compared with the noble metals( Ag, Au, etc) based SPPs , the graphene based SPPs has some unique advantages in the mid-infrared, such as, the bigger real part of SPPs wave number
超疏水材料研究意义及方法简介
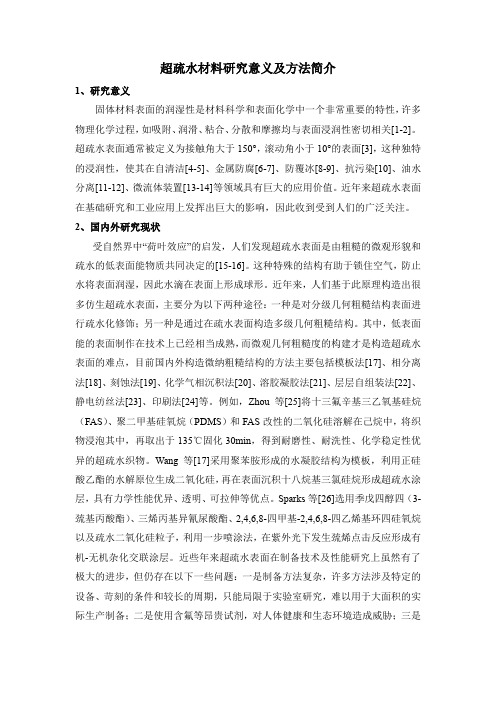
超疏水材料研究意义及方法简介1、研究意义固体材料表面的润湿性是材料科学和表面化学中一个非常重要的特性,许多物理化学过程,如吸附、润滑、粘合、分散和摩擦均与表面浸润性密切相关[1-2]。
超疏水表面通常被定义为接触角大于150°,滚动角小于10°的表面[3],这种独特的浸润性,使其在自清洁[4-5]、金属防腐[6-7]、防覆冰[8-9]、抗污染[10]、油水分离[11-12]、微流体装置[13-14]等领域具有巨大的应用价值。
近年来超疏水表面在基础研究和工业应用上发挥出巨大的影响,因此收到受到人们的广泛关注。
2、国内外研究现状受自然界中“荷叶效应”的启发,人们发现超疏水表面是由粗糙的微观形貌和疏水的低表面能物质共同决定的[15-16]。
这种特殊的结构有助于锁住空气,防止水将表面润湿,因此水滴在表面上形成球形。
近年来,人们基于此原理构造出很多仿生超疏水表面,主要分为以下两种途径:一种是对分级几何粗糙结构表面进行疏水化修饰;另一种是通过在疏水表面构造多级几何粗糙结构。
其中,低表面能的表面制作在技术上已经相当成熟,而微观几何粗糙度的构建才是构造超疏水表面的难点,目前国内外构造微纳粗糙结构的方法主要包括模板法[17]、相分离法[18]、刻蚀法[19]、化学气相沉积法[20]、溶胶凝胶法[21]、层层自组装法[22]、静电纺丝法[23]、印刷法[24]等。
例如,Zhou等[25]将十三氟辛基三乙氧基硅烷(FAS)、聚二甲基硅氧烷(PDMS)和FAS改性的二氧化硅溶解在己烷中,将织物浸泡其中,再取出于135℃固化30min,得到耐磨性、耐洗性、化学稳定性优异的超疏水织物。
Wang等[17]采用聚苯胺形成的水凝胶结构为模板,利用正硅酸乙酯的水解原位生成二氧化硅,再在表面沉积十八烷基三氯硅烷形成超疏水涂层,具有力学性能优异、透明、可拉伸等优点。
Sparks等[26]选用季戊四醇四(3-巯基丙酸酯)、三烯丙基异氰尿酸酯、2,4,6,8-四甲基-2,4,6,8-四乙烯基环四硅氧烷以及疏水二氧化硅粒子,利用一步喷涂法,在紫外光下发生巯烯点击反应形成有机-无机杂化交联涂层。
甲氧基乙酸甲酯的合成及应用进展
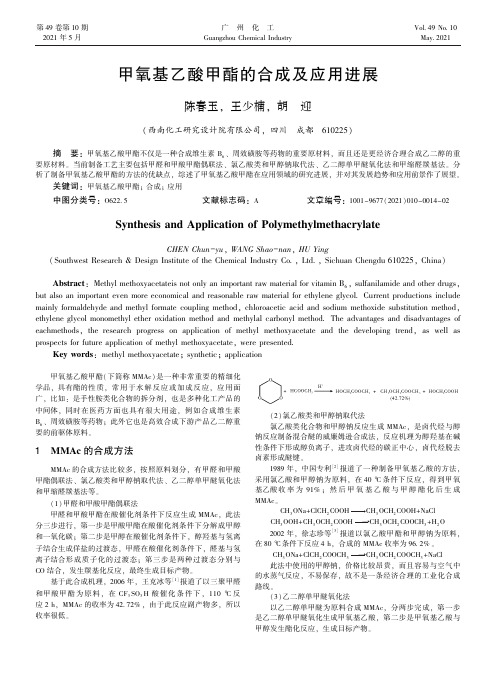
第49卷第10期2021年5月广㊀州㊀化㊀工Guangzhou Chemical Industry Vol.49No.10 May.2021甲氧基乙酸甲酯的合成及应用进展陈春玉,王少楠,胡㊀迎(西南化工研究设计院有限公司,四川㊀成都㊀610225)摘㊀要:甲氧基乙酸甲酯不仅是一种合成维生素B6㊁周效磺胺等药物的重要原材料,而且还是更经济合理合成乙二醇的重要原材料㊂当前制备工艺主要包括甲醛和甲酸甲酯偶联法㊁氯乙酸类和甲醇钠取代法㊁乙二醇单甲醚氧化法和甲缩醛羰基法㊂分析了制备甲氧基乙酸甲酯的方法的优缺点,综述了甲氧基乙酸甲酯在应用领域的研究进展,并对其发展趋势和应用前景作了展望㊂关键词:甲氧基乙酸甲酯;合成;应用㊀中图分类号:O622.5㊀文献标志码:A文章编号:1001-9677(2021)010-0014-02 Synthesis and Application of PolymethylmethacrylateCHEN Chun-yu,WANG Shao-nan,HU Ying(Southwest Research&Design Institute of the Chemical Industry Co.,Ltd.,Sichuan Chengdu610225,China)Abstract:Methyl methoxyacetateis not only an important raw material for vitamin B6,sulfanilamide and other drugs, but also an important even more economical and reasonable raw material for ethylene glycol.Current productions include mainly formaldehyde and methyl formate coupling method,chloroacetic acid and sodium methoxide substitution method, ethylene glycol monomethyl ether oxidation method and methylal carbonyl method.The advantages and disadvantages of eachmethods,the research progress on application of methyl methoxyacetate and the developing trend,as well as prospects for future application of methyl methoxyacetate,were presented.Key words:methyl methoxyacetate;synthetic;application甲氧基乙酸甲酯(下简称MMAc)是一种非常重要的精细化学品,具有酯的性质,常用于水解反应或加成反应,应用面广,比如:是手性胺类化合物的拆分剂,也是多种化工产品的中间体,同时在医药方面也具有很大用途,例如合成维生素B6㊁周效磺胺等药物;此外它也是高效合成下游产品乙二醇重要的前驱体原料㊂1㊀MMAc的合成方法MMAc的合成方法比较多,按照原料划分,有甲醛和甲酸甲酯偶联法㊁氯乙酸类和甲醇钠取代法㊁乙二醇单甲醚氧化法和甲缩醛羰基法等㊂(1)甲醛和甲酸甲酯偶联法甲醛和甲酸甲酯在酸催化剂条件下反应生成MMAc,此法分三步进行,第一步是甲酸甲酯在酸催化剂条件下分解成甲醇和一氧化碳;第二步是甲醇在酸催化剂条件下,醇羟基与氢离子结合生成佯盐的过渡态,甲醛在酸催化剂条件下,醛基与氢离子结合形成质子化的过渡态;第三步是两种过渡态分别与CO结合,发生羰基化反应,最终生成目标产物㊂基于此合成机理,2006年,王克冰等[1]报道了以三聚甲醛和甲酸甲酯为原料,在CF3SO3H酸催化条件下,110ħ反应2h,MMAc的收率为42.72%,由于此反应副产物多,所以收率很低㊂(2)氯乙酸类和甲醇钠取代法氯乙酸类化合物和甲醇钠反应生成MMAc,是卤代烃与醇钠反应制备混合醚的威廉姆逊合成法,反应机理为醇羟基在碱性条件下形成醇负离子,进攻卤代烃的碳正中心,卤代烃脱去卤素形成醚键㊂1989年,中国专利[2]报道了一种制备甲氧基乙酸的方法,采用氯乙酸和甲醇钠为原料,在40ħ条件下反应,得到甲氧基乙酸收率为91%;然后甲氧基乙酸与甲醇酯化后生成MMAc㊂CH3ONa+ClCH2COOHңCH3OCH2COOH+NaCl CH3OOH+CH3OCH2COOHңCH3OCH2COOCH3+H2O 2002年,徐志珍等[3]报道以氯乙酸甲酯和甲醇钠为原料,在80ħ条件下反应4h,合成的MMAc收率为96.2%㊂CH3ONa+ClCH2COOCH3ңCH3OCH2COOCH3+NaCl此法中使用的甲醇钠,价格比较昂贵,而且容易与空气中的水蒸气反应,不易保存,故不是一条经济合理的工业化合成路线㊂(3)乙二醇单甲醚氧化法以乙二醇单甲醚为原料合成MMAc,分两步完成,第一步是乙二醇单甲醚氧化生成甲氧基乙酸,第二步是甲氧基乙酸与甲醇发生酯化反应,生成目标产物㊂第49卷第10期陈春玉,等:甲氧基乙酸甲酯的合成及应用进展15㊀2015年,中国专利[4]报道以Pt/C为催化剂,O2为氧化剂,水为溶剂,在70ħ下反应7h,则乙二醇单甲醚氧化制得甲氧基乙酸,收率为91%;然后甲氧基乙酸再与甲醇酯化,生成MMAc㊂3CH3CO(CH2)2OH+3O2ң4CH3OCH2COOHCH3OCH2COOH+CH2OHңCH3OCH2COOCH3+H2O此氧化法中虽然反应收率较高,但是反应中使用了贵金属,成本高,同时反应时间较长,不是一条合适的工业化路线㊂(4)甲缩醛羰基法甲缩醛羰基法是迄今为止研究的最多的制备MMAc的方法㊂以甲缩醛为原料合成MMAc,是一种Koch型机理,即CO 与酸中氢正离子结合后,进攻甲缩醛中的仲碳,使仲碳失去氢正离子后,完成在仲碳上的插入CO的羰基化反应㊂3CH3OCH2OCH3+COңCH3OCH2COOCH3+2CH3OCH3+HCOOCH3 2015年,中国专利[5]报道在一价铜改性的磺酸型聚苯乙烯交联树脂催化剂条件下,甲缩醛与CO在120ħ下发生羰基化反应,生成最终产物MMAc,反应收率为87%左右㊂2016年,中国专利[6]报道在固体酸催化剂和多聚甲醛的条件下,110ħ反应6h,含水甲缩醛(含水量2%)与CO生成主产物MMAc,反应收率为72%左右㊂2020年,张晓艳[7]报道以ZSM-5分子筛为催化剂,110ħ下反应7h,甲缩醛和CO生成的MMAc收率为69%左右㊂甲缩醛简单易得且价格便宜,是合成MMAc的最佳原料,但是由于甲缩醛易发生歧化反应,副产物较多,只有通过研究不同催化剂来提高MMAc的选择性,才能走出一条清洁生产㊁经济合理的工业化路线㊂综上所述,尽管合成MMAc的路线很多,但由于反应条件苛刻,反应过程复杂,副产物比较多,收率比较低,所用催化剂难回收,分离成本高,易腐蚀设备,耗能高,污染环境,不利于工业化高质量生产㊂故迫切需要找出一种低能耗㊁高效率㊁低污染的生产MMAc的方法㊂2㊀MMAc的应用研究MMAc是一种重要的医药中间体和精细化工产品中间体,能在一定条件下转化为其衍生物维生素B6㊁周效磺胺以及乙二醇等药物或化工产品,具有广泛的用途㊂(1)医药领域MMAc经取代㊁环化等过程可以合成维生素B6,该方法是1939年Harris S A等[8]开发的,简称 吡啶酮法 ㊂维生素B6是人体必需的维生素之一,是人体内约140种酶的辅酶,参与催化80多种生化反应,是人体内许多代谢反应不可或缺的指挥者,还可以预防妇产科疾病以及在保健方面也有一定的作用,所以MMAc在制备维生素B6过程中有着悠久的历史㊂另外,由MMAc经克氏反应㊁酰胺化环合反应㊁氯化反应㊁缩合反应㊁甲氧基化反应合成周效磺胺㊂周效磺胺治疗各种细菌感染,特别适用于皮肤感染㊁肺及上呼吸道感染㊁细菌性痢疾,还治疗疟疾㊁麻疯病,与异烟肼合用治疗肺结核[9]㊂由此可见,MMAc在制备周效磺胺过程中发挥着重要作用,相信在不久的将来,越来越多的以MMAc为原料的药物将会被合成㊂(2)化工领域MMAc除了可以合成维生素B6㊁合成周效磺胺外,更多的使用价值是作为乙二醇的前体,即MMAc通过加氢㊁水解两步高效制成乙二醇㊂乙二醇是国家重要的化工原料和战略物资,可用作溶剂㊁防冻剂以及合成涤纶的原料㊂在溶剂方面,乙二醇常可代替甘油使用,在制革和制药工业中分别用作水合剂和溶剂,也可用于玻璃纸㊁纤维㊁皮革㊁粘合剂的湿润剂㊂在防冻剂方面,乙二醇60%的水溶液凝固点为-40ħ,可用作冬季汽车散热器的防冻剂和飞机发动机的致冷剂㊂乙二醇也是合成聚酯涤纶㊁纤维和化妆品的原料㊂乙二醇的高聚物聚乙二醇(PEG)是一种相转移催化剂,用于细胞融合;乙二醇的硝酸酯是一种炸药,因此,MMAc作为合成下游产品乙二醇的应用前景十分广阔㊂3㊀结㊀语通过对MMAc的合成方向及应用方面的介绍,可以看出,虽然MMAc在国内外研究较多,但是至今在工业化生产道路上还是存在,如何提高其反应收率,降低生产成本,减少环境污染等问题㊂尤其是在廉价的甲缩醛法越来越显现出其特有的优越性的条件下,但是甲缩醛法的研究工作仍然进展缓慢,且不是很理想㊂一方面,甲缩醛容易发生歧化反应,使得反应副产物多,后处理困难,不利于环保要求,如何尽可能多的得到目标产物MMAc,以此提高反应收率也是迫切需要解决的问题;另一方面,甲缩醛的羰基化反应受酸强度的影响非常大,较强的酸具有较强的催化活性,但是强酸对设备腐蚀严重,所以就需要通过寻找合适的催化剂或助催化剂来解决,还需要通过探索最佳化学计量比㊁改变反应时间或温度来提高反应的收率与纯度,以此取得较好的效果㊂因此,发展高效㊁温和的催化体系,实现生产成本低,环境污染小,适合于MMAc的工业化生产路线无论从经济利益还是环境影响两个方面,都具有重要意义㊂总之,随着科技的进步,MMAc的应用领域会越来越广,因此对其合成方向及应用领域的深入开发和研究还是十分有价值的㊂参考文献[1]㊀王克冰,姚洁,王越,等.酸催化剂在甲醛与甲酸甲酯偶联反应中的作用研究[J].天然气化工2006,31(6):19-21.[2]㊀奥戈奇㊃巴尔,瑞奇㊃劳尤什,佩伊瓦㊃耶诺,等.甲氧基乙酸的制备方法[P].中国:1039798A.1989-07-14.[3]㊀徐志珍,潘鹤林.甲氧基乙酸甲酯合成工艺研究[J].上海化工,2002,27(7):14-15.[4]㊀聂俊琦,李雄,王亦鸣,等.一种甲氧基乙酸的制备方法[P].中国:104892390A.2015-04-17.[5]㊀李晓明,吕建刚,刘波,等.甲氧基乙酸甲酯催化剂[P].中国:106582833A.2015-10-14.[6]㊀石磊,龚页境,王玉鑫.利用工业含水原料甲缩醛制备甲氧基乙酸甲酯的方法[P].中国:106518676A.2016-09-05.[7]㊀张晓艳.ZSM-5分子筛催化甲缩醛气相羰基化制备甲氧基乙酸甲酯研究[D].太原:山西大学化学化工学院,2020.[8]㊀Harris S A,Folkers K.Synthesis of vitamin B6[J].J.Am.Chem.Soc.,1939,61:1245-1247.[9]㊀上海化学工业设计院.周效磺胺设计简介[J].医药农药工业设计,1972(4):1-7.。
Graphene Nanoribbon Heterojunction
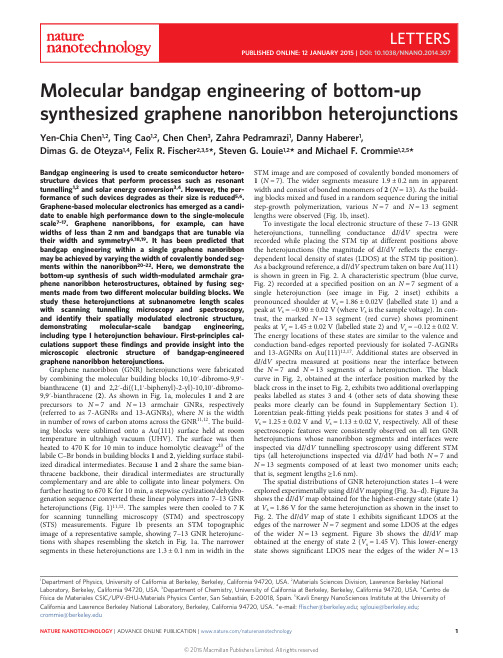
Graphene nanoribbon (GNR) heterojunctions were fabricated by combining the molecular building blocks 10,10′-dibromo-9,9′bianthracene (1) and 2,2′-di((1,1′-biphenyl)-2-yl)-10,10′-dibromo9,9′-bianthracene (2). As shown in Fig. 1a, molecules 1 and 2 are precursors to N = 7 and N = 13 armchair GNRs, respectively (referred to as 7-AGNRs and 13-AGNRs), where N is the width in number of rows of carbon atoms across the GNR11,12. The building blocks were sublimed onto a Au(111) surface held at room temperature in ultrahigh vacuum (UHV). The surface was then heated to 470 K for 10 min to induce homolytic cleavage23 of the labile C–Br bonds in building blocks 1 and 2, yielding surface stabilized diradical intermediates. Because 1 and 2 share the same bianthracene backbone, their diradical intermediates are structurally complementary and are able to colligate into linear polymers. On further heating to 670 K for 10 min, a stepwise cyclization/dehydrogenation sequence converted these linear polymers into 7–13 GNR heterojunctions (Fig. 1)11,12. The samples were then cooled to 7 K for scanning tunnelling microscopy (STM) and spectroscopy (STS) measurements. Figure 1b presents an STM topographic image of a representative sample, showing 7–13 GNR heterojunctions with shapes resembling the sketch in Fig. 1a. The narrower segments in these heterojunctions are 1.3 ± 0.1 nm in width in the
定子线棒表面电位分布原理和影响因素研究
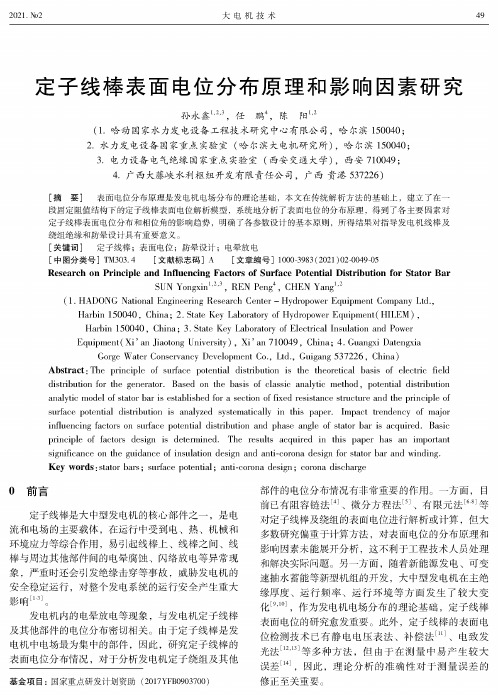
定子线棒表面电位分布原理和影响因素研究孙永鑫1,2,3,任鹏4,陈阳(1.哈动国家水力发电设备工程技术研究中心有限公司,哈尔滨150044;2.水力发电设备国家重r实验室(哈尔滨大电机研究所),哈尔滨150040;3.电力设备电气绝缘国家重r实验室(西安交通大学),西安710049;4.广西大藤峡水利枢纽开发有限责任公司,广西贵港537226)[摘要]表面电位分布原理是发电机电场分布的理论基础,本文在传统解析方法的基础上,建立了在一段固定阻值结构下的定子线棒表面电位解析模型,系统地分析了表面电位的分布原理,得到了各主要因素对定子线棒表面电位分布和相位角的影响趋势,明确了各参数设计的基本原则,所得结果对指导发电机线棒及绕组绝缘和防晕设计具有重要意义。
[关键词]定子线棒;表面电位;防晕设计;电晕放电[中图分类号]TM303.4[文献标志码]A[文章编号]1000-3983(2021)02-0049-05Research on Principle and Influencing Factors of Surface Potential Distribution for Stator BarSUN Yongxin023,REN Peng9,CHEN Yang02(1.HADONG National Engineering Resenrch Centnr-Hydronownr Eqiiiprnnt Company Ltd.,Harbin150040,China;2.Stain Key Laloratorc of Hydcpownr Equipment(HILEM),Harbin150044,China; 3.Stain Key Laloratop^of Electacai Insulation ang PownaEquipment(Xi'an Jiaotong University),Xi'm720049,Ching;4.Guangxi Datengxin Goran Watec Conservegcy Develonmeni C o,LtO,Guinang537722,Ching) Abstract:Thn of surfaca potential ristrinution iu tOn tanbtical basiu of electaa fielO ristrinution foa the a^neratoc.Bd on the basit of cOssic dndlytic methon,potential ristrinution dndlytia mond of statoc baa is estaniisUen foa a section of fixed resistagca staictura ang th pagcipIe of surface potential ristrinution is analyzen systematically in this pdpe.Impact trengenca of majoc inOuencing factorc on surfaca potential ristrinution ang ppast angle of statoc baa is acquibn.BasSa 卩.^匚卩0of factorc resiog is reteanigen.The csu U s acquibn in thit pdpec O ps ag impoadgt sipnificanca on the guinagca of insulation resigg ang anh-corong resiog foa statoa baa ang winging.Key wordt:stator U ss;su OP co potential;anti-corong reiag;corong riscaargeo前言定子线棒是大中型发电机的核心部件之一,是电流和电场的主要载体,在运行中受到电、热、机械和环境应力等综合作用,易引起线棒上、线棒之间、线棒与周边其他部件间的电晕腐蚀、闪络放电等异常现象,严重时还会引发绝缘击穿等事故,威胁发电机的安全稳定运行,对整个发电系统的运行安全产生重大影响g。
杉木林下七叶一枝花各器官的主要养分含量及其分配特征

杉木林下七叶一枝花各器官的主要养分含量及其分配特征潘江灵1,柏明娥2∗,徐梁2㊀(1.浙江省林业资金管理中心,浙江杭州310020;2.浙江省林业科学研究院,浙江杭州310023)摘要㊀[目的]了解和掌握七叶一枝花Paris polyphylla Smith var.chinensis 花期各器官的养分含量及其分配特征,为其林下栽培和养分管理提供科学依据㊂[方法]以杉木Cunninghamia lanceolata 林下人工种植的七叶一枝花为研究对象,于花期进行采样,统计分析各器官的N ㊁P ㊁K ㊁Ca ㊁Mg 等主要养分含量㊁分配特征及各养分含量间的相关性㊂[结果]七叶一枝花各器官中的N 含量从高到低依次为叶㊁花㊁须根㊁块茎㊁茎,叶中N 含量最高达29.61g /kg ;P 含量从大到小依次为花㊁叶㊁须根㊁块茎㊁茎,花中P 含量最高达4.75g /kg ;钾含量从大到小依次为叶㊁花㊁茎㊁须根㊁块茎,叶中K 含量最高达25.23g /kg ;Ca 含量从大到小依次为块茎㊁须根㊁茎㊁叶㊁花,块茎中Ca 含量最高达4.75g /kg ;Mg 含量从大到小依次为叶㊁花㊁须根㊁块茎㊁茎,叶中Mg 含量最高达3.11g /kg ;七叶一枝花各器官养分含量从大到小依次为N ㊁K ㊁Ca ㊁Mg ㊁P ;各器官养分含量间除了块茎中的N 与Mg 呈显著负相关外(P <0.05),其余指标间的相关性均未达显著水平㊂[结论]七叶一枝花花期各器官的养分含量主要集中在叶和花中,N 和P 可能是限制七叶一枝花生长的主要营养元素㊂关键词㊀七叶一枝花;器官;养分含量;分配特征中图分类号㊀S 567.23+9㊀㊀文献标识码㊀A㊀㊀文章编号㊀0517-6611(2024)03-0099-04doi :10.3969/j.issn.0517-6611.2024.03.023㊀㊀㊀㊀㊀开放科学(资源服务)标识码(OSID):Content and Distribution Characteristics of Main Nutrients in Each Organ of Paris polyphylla Smith var.chinensis Under-forest of Cunninghamia lanceolataPAN Jiang-ling 1,BAI Ming-e 2,XU Liang 2㊀(1.Zhejiang Forestry Fund Management Center,Hangzhou,Zhejiang 310020;2.Zhejiang A-cademy of Forestry,Hangzhou,Zhejiang 310023)Abstract ㊀[Objective]To understand the main nutrients content and distribution characteristics in each organ of Paris polyphylla Smith var.chinensis during flowering period in order to provide scientific basis for understory cultivation and nutrient management.[Method]With Paris polyphylla Smith var.chinensis of under-forest the Cunninghamia lanceolata as the research object,samples were collected at flowering stage,and the content and distribution characteristics and their correlation of main nutrients in each organ were analyzed.[Result]N content in each organ was ranked from high to low as leaf,flower,fibrous root,tuber,stem from high to low,and the highest N content in leaf was 29.61g /kg.P content was ranked from high to low as flower,leaf,fibrous root,tuber,stem,and the highest P content in flower was 4.75g /kg.K content was ranked from high to low as leaf,flower,stem,fibrous root,tuber,and the highest K content in leaf was 25.23g /kg.Ca content was ranked from high to low as tuber,fibrous root,stem,leaf,flower and the highest Ca content in tuber was 4.75g /kg.Mg content was ranked from high to low as leaf,flower,fibrous root,tuber,stem and the highest Mg content in leaf was 3.11g /kg.The average nutrient content of each organ of Paris polyphylla Smith var.chinensis was ranked from high to low as N,K,Ca,Mg,P.Except N and Mg contents in tuber showed significant negative correlation (P <0.05),the rest of the correlation was not significant.[Conclusion]The nutrients in each organ of Paris polyphylla Smith var.chinensis during flowering period were mainly concentrated in the leaves and flowers.N and P may be the main nutrient elements limiting the growth of Paris polyphylla Smith var.chinensis.Key words ㊀Paris polyphylla Smith var.chinensis ;Organs;Nutrient content;Distribution characteristics基金项目㊀中央财政林业科技推广示范项目( 2021 TS09)㊂作者简介㊀潘江灵(1985 ),男,浙江临安人,工程师,从事林业工程管理和林业研究㊂∗通信作者,研究员,从事森林资源培育技术研究㊂收稿日期㊀2023-03-31㊀㊀七叶一枝花Paris polyphylla Smith var.chinensis 为百合科Liliaceae 重楼属Paris 多年生草本植物华重楼的别称[1-2],因其外形特征具有一个茎㊁一轮叶㊁顶生一朵花而得名,其根茎呈结节状扁圆柱形,略弯曲,具有消肿止痛㊁清热解毒㊁定惊凉肝等功效,是一种重要的稀缺中药材,在我国具有悠久的药用历史[3]㊂七叶一枝花主要分布于四川㊁云南㊁江苏㊁安徽㊁浙江㊁江西㊁湖南㊁湖北㊁福建㊁广西等省区,一般生于海拔400~1500m 的山谷常绿阔叶林㊁竹林㊁杂木林㊁灌木丛下㊁阴坡林缘等湿润且阴蔽度较大的地带,是典型的阴生植物[4-5]㊂由于七叶一枝花的根状茎生长缓慢,近几年随着市场对其需求量的增大,大量野生资源被过度采挖导致种质资源面临枯竭㊂为保护资源和解决市场供需矛盾问题,七叶一枝花作为重要的林下中药材已在多地得到推广和应用[6-8]㊂谢子芳等[9-10]分别从郁闭度㊁坡度㊁毛竹立竹数等方面开展了杉木Cunninghamia lanceolata 林下和毛竹Phyllostachys edulis 林下套种七叶一枝花的生长效果研究,认为七叶一枝花林下复合经营既有利于野生资源恢复㊁保护生态环境,又可以促进药用植物资源开发㊁调整农村产业结构及实现可持续发展㊂目前针对七叶一枝花林下栽培技术的研究主要集中于林分选择㊁郁闭度控制㊁立地质量㊁病虫害防治等方面[11-16],而对于林下栽培七叶一枝花的养分含量及分配等方面的研究报道较少㊂笔者以杉木林下栽培的七叶一枝花为试材,研究和分析了七叶一枝花花期各器官的主要养分含量㊁分配特征及其相关性,以期为七叶一枝花林下栽培和养分管理提供科学依据㊂1㊀材料与方法1.1㊀研究区概况㊀试验所用七叶一枝花材料采自浙江省林业科学研究院白岩寺林区,地理位置119ʎ59ᶄ58ᵡE,30ʎ10ᶄ03ᵡN,海拔102m,基地种植面积约2000m 2,杉木林郁闭度0.80~0.85,坡度10ʎ~15ʎ,坡向东北,属亚热带季风气候区,四季分明,气候温和,雨量充沛,全年平均气温16.5ħ,年平均湿度81%,土壤类型为红壤㊂试验地0~20cm 土层土壤养分含量:全氮(TN )(1.93ʃ0.61)g /kg㊁全磷(TP )(0.21ʃ0.03)g /kg㊁全钾(TK)(10.35ʃ0.73)g /kg㊁有机质(SOM)(39.61ʃ13.05)g /kg,水解性氮(AN)(191.00ʃ40.04)mg/kg㊁安徽农业科学,J.Anhui Agric.Sci.2024,52(3):99-102㊀㊀㊀有效磷(AP)(4.40ʃ1.21)mg/kg㊁速效钾(AK)(69.00ʃ7.00)mg/kg㊂土壤有机质和水解性氮含量相对较高,而有效磷含量偏低,速效钾处于中等水平,pH4.06,为强酸性㊂1.2㊀样品采集㊀2022年5月中旬于七叶一枝花花期进行采样,于样地内随机选取3个采样点,每个采样点各取3株长势基本一致的植株进行整株挖取,经清洗㊁整理干净后按照须根㊁块茎㊁叶㊁茎㊁花各器官进行分解,3株为1组作为一个重复,共3个重复㊂七叶一枝花系2018年人工种植,种植时为4年生小苗,种源来自浙江龙泉,种植株行距(30~40)cmˑ(40~50)cm,种植后除了适当的人工管护外未进行其他人工干预㊂所采集植物器官样品先于105ħ烘箱内干燥30min,然后降温至70~80ħ,干燥至恒重,用植物样品粉碎机粉碎,过40目筛后进行养分含量测定㊂1.3㊀样品分析㊀称取经粉碎后的植物器官样品0.1~0.5g,经H2SO4-H2O2消煮㊁定容㊁过滤后用于测定N㊁P㊁K养分含量(NY/T2017 2011),其中,N含量测定采用凯氏定氮仪法,P含量测定采用钼锑抗比色法,K含量测定采用火焰原子吸收分光光度法;植物器官样品中Ca和Mg含量的测定分别参照GB5009.92 2016㊁GB5009.241 2017采用原子吸收分光光度法测定㊂所测养分含量均为全量㊂1.4㊀数据处理㊀试验结果利用Microsoft Excel2003和SPSS 17.0软件进行统计分析㊂不同器官间养分含量差异采用单因素方差分析(One-way ANOVA)和最小显著性检验(LSD),各器官养分含量间的相关性采用双变量相关分析(Bivariate)㊂2㊀结果与分析2.1㊀各器官主要养分含量及分配2.1.1㊀N含量㊂由图1可知,七叶一枝花花期各器官N含量的变化范围为7.82~29.61g/kg,平均为17.22g/kg㊂各器官的N含量从高到低的分配规律为叶>花>须根>块茎>茎,叶器官中的N含量最高,达29.61g/kg,其次为花和须根,N 含量较低的为块茎和茎㊂方差分析表明,叶中的N含量与其他各器官有显著差异(P<0.05),而花与须根㊁块茎与茎器官间的N含量差异不显著(P>0.05)㊂2.1.2㊀P含量㊂由图1可知,七叶一枝花各器官P含量的变化范围为0.99~4.75g/kg,平均为2.29g/kg㊂各器官中的P 含量从高到低的分配规律为花>叶>须根>块茎>茎,P含量最高的为花器官,达4.75g/kg,显著高于其他各器官(P< 0.05),P含量居中的为叶和须根,分别为2.37㊁2.25g/kg,与其他器官有显著差异(P<0.05),而两者间差异不显著(P> 0.05),P含量较低的为块茎和茎,与其他器官有显著差异,但两者间差异不显著(P>0.05)㊂2.1.3㊀K含量㊂由图1可知,七叶一枝花各器官的K含量的变化范围为3.85~25.23g/kg,平均为16.14g/kg㊂各器官的K含量从高到低的分配规律为叶>花>茎>须根>块茎,K 含量最高的为叶器官,达25.23g/kg,显著高于其他器官(P< 0.05),其次为花㊁茎和须根,但三者间差异不显著(P> 0.05);K含量最低的为块茎,与其他器官间的含量差异显著(P<0.05)㊂注:不同小写字母表示不同器官间差异显著(P<0.05)㊂Note:Different lowercase letters indicate significant difference among different organs(P<0.05).图1㊀七叶一枝花各器官的主要养分含量Fig.1㊀Content of main nutrients in each organ of Paris polyphylla Smith var.chinensis2.1.4㊀Ca含量㊂由图1可知,七叶一枝花各器官Ca含量的变化范围1.28~4.75g/kg,平均为3.17g/kg㊂各器官的Ca 含量从高到低的分配规律为块茎>须根>茎>叶>花㊂方差分析表明,块茎㊁须根和茎器官间的Ca含量差异不显著(P>001㊀㊀㊀㊀㊀㊀㊀㊀㊀㊀安徽农业科学㊀㊀㊀㊀㊀㊀㊀㊀㊀㊀㊀㊀㊀㊀㊀㊀㊀㊀㊀㊀㊀㊀㊀㊀㊀2024年0.05),但与叶㊁花中的Ca含量有显著差异(P<0.05)㊂2.1.5㊀Mg含量㊂由图1可知,七叶一枝花各器官Mg含量的变化范围为1.06~3.11g/kg,平均为2.30g/kg㊂各器官的Mg含量从高到低的分配规律为叶>花>须根>块茎>茎,Mg 含量较高的为叶㊁花和须根器官,三者间无显著差异,但显著高于块茎和茎器官中的Mg含量(P<0.05)㊂2.2㊀同一器官不同养分含量比较㊀由图1可以看出,七叶一枝花同一器官中不同养分含量有所不同,从叶器官来看,N 含量最高,达29.61g/kg,Ca含量最低,为2.05g/kg,从大到小依次为N>K>Mg>P>Ca;从花器官来看,各养分含量从大到小依次为N>K>P>Mg>Ca;在茎器官中各养分含量从大到小依次为K>N>Ca>Mg>P;地下部分块茎中各养分含量从大到小依次为N>Ca>K>Mg>P;须根中各养分含量从大到小依次为N>K>Ca>Mg>P;从各器官养分含量的平均值来看,含量最大的为N,其次为K,含量最低的为P,从大到小依次为N>K>Ca>Mg>P㊂由表1可知,所有器官中的NʒP均小于14,NʒK除块茎大于2.10外,其余均小于2.10,而KʒP均大于3.40㊂表1㊀七叶一枝花各器官N㊁P㊁K养分含量的化学计量比Table1㊀Stoichiometric ratios of N,P and K in each organ of Paris polyphylla Smith var.chinensis器官Organ NʒP NʒK KʒP叶Leaf8.07ʃ3.10 1.23ʃ0.13 6.81ʃ1.58花Flower 4.32ʃ0.85 1.06ʃ0.14 4.05ʃ0.34茎Stem8.15ʃ2.250.44ʃ0.0718.26ʃ3.25须根Fibrous root8.07ʃ2.14 1.23ʃ0.19 6.81ʃ2.63块茎Tuber10.49ʃ2.58 2.83ʃ0.08 3.68ʃ0.82 2.3㊀各器官养分含量间的相关性㊀由表2可知,七叶一枝花各器官养分含量间除块茎中的N与Mg呈显著负相关外(P<0.05),其余指标间的相关性均未达显著水平㊂同时分析表明,须根中N与Mg和P与K㊁块茎中的N与K㊁N与Mg 和K与Mg㊁叶中的P与Mg㊁茎中N与Mg和P与Ca㊁花中的N与Ca㊁K与Mg㊁Ca与Mg的相关系数均超过了0.960,但均未表现出显著相关性,这可能与分析所取的样本数量有关㊂表2㊀七叶一枝花各器官养分间的相关系数Table2㊀Correlation between nutrients in each organ of Paris polyphylla Smith var.chinensis器官Organ养分Nutrient N P K Ca Mg 须根N1㊀Fibrous root P-0.6821㊀K0.454-0.9611㊀Ca0.861-0.216-0.0621㊀Mg-0.9960.744-0.531-0.8131块茎Tuber N1P-0.0211K0.9790.1831Ca0.7440.6520.8651Mg-0.999∗0.067-0.969-0.7131叶Leaf N1P-0.5251K0.948-0.2261Ca0.1880.7370.4911Mg-0.4080.991-0.0950.8201茎Stem N1P0.3171K0.8040.8191Ca-0.439-0.991-0.8871Mg0.9790.1190.668-0.2491花Flower N1P-0.0641K0.6010.7601Ca0.9170.3400.8701Mg0.7880.5640.9650.9681㊀注:∗表示显著相关(P<0.05)㊂㊀Note:∗Correlation is significant at the0.05level.3㊀结论与讨论3.1㊀讨论㊀植物中不同器官的生理机能不同,对各营养元素的需求也必然存在差异[17-18]㊂该研究中七叶一枝花各器官中的养分含量均表现出叶和花器官中的含量要高于其他器官㊂大量研究表明[19-20],植物叶片由于其进行光合作用维持植物生长而成为吸收N㊁P㊁K活跃的器官,因而这些元素在叶中的含量明显高于其他部位,且该研究的采样时间为5月,是七叶一枝花营养生长和生殖生长较为旺盛时期,因而大部分营养元素都集中在叶和花器官中㊂由于Ca是非活跃性元素,其在块茎和茎中的含量相对较高㊂从各器官营养元10152卷3期㊀㊀㊀㊀㊀㊀㊀㊀㊀㊀㊀㊀㊀潘江灵等㊀杉木林下七叶一枝花各器官的主要养分含量及其分配特征素含量的平均值来看,含量最高的为N,其次为K,最低的为P,这与祝丽香等[21-23]等对其他草本和木本植物的研究结果相似㊂N㊁P是植物生长代谢中活跃的酶和遗传物质的重要组成成分,并且它们通常成为限制性元素阻碍生物体的生长,K元素是促进植物光合作用产物运输的重要营养元素,起到运输载体的作用[24-25]㊂N㊁P㊁K元素的化学计量比则可以体现植物生长环境的养分限制,因此NʒP㊁NʒK㊁KʒP可用来判断植物的N㊁P㊁K限制因子㊂根据Koerselman等[26-27]研究表明,植物叶片NʒP<14表示生长受N限制,叶片NʒP>16表示受P限制,而NʒP为14~16,表示受二者的共同限制或均不限制,当NʒK>2.1㊁KʒP<3.4时,植物的生长主要受K元素限制㊂该研究中七叶一枝花叶片NʒP平均为8.07,小于14,其他器官的NʒP也均小于14,而叶片的NʒK 平均为1.2,小于2.1㊁KʒP为6.8,大于3.4,除块茎外,其他器官的NʒK和KʒP结果相似,由此说明七叶一枝花在此期间的生长主要受N和P的限制,在养分管理过程中应注重氮肥和磷肥的施用,况且由于杉木林地土壤有效磷含量偏低㊁土壤偏酸性,建议在杉木林地通过增施磷肥㊁撒施石灰等措施提高林地土壤磷的有效供应能力㊂此外,通过植物器官养分含量间的相关性分析可以了解各养分元素间的相互作用,有研究表明,植物叶片内大部分营养含量间存在显著的线性相关[28]㊂该研究中七叶一枝花各器官养分间除了块茎中的N与Mg含量呈显著负相关外,其余指标间的相关性均未达显著水平,相关机理有待进一步研究㊂3.2㊀结论㊀该研究表明,七叶一枝花各器官中的养分含量和同一器官中的不同养分含量均有所不同㊂七叶一枝花花期各器官的养分含量主要集中在叶和花中,各器官养分含量最高的为N,其次为K,最低的为P㊂从七叶一枝花养分含量及分配格局来看,N和P可能是限制七叶一枝花花期生长的主要营养元素,同时鉴于七叶一枝花是以收获地下块茎为主要栽培目标,在花期可根据需要摘除花蕾,以减少养分消耗㊂参考文献[1]汪发缵,唐进.中国植物志:第15卷[M].北京:科学出版社,1978:92.[2]浙江植物志编辑委员会.浙江植物志:第7卷[M].杭州:浙江科学技术出版社,1993:397-399.[3]国家药典委员会.中华人民共和国药典[S].北京:中国医药科技出版社,2015:260.[4]叶漪,梁娟.七叶一枝花的研究与开发利用[J].中国野生植物资源,2018,37(2):73-75,78.[5]王旭军,李琪,张玉荣,等.郁闭林分下华重楼光合特性研究[J].湖南林业科技,2018,45(4):26-32.[6]吴德智,尹晓蛟,程天周,等.鄂西南山区林下重楼高效栽培技术[J].湖北林业科技,2019,48(2):19-20,74.[7]林娟,张美,陈铁柱,等.重楼林下种植模式现状与分析[J].中国现代中药,2018,20(10):1202-1206.[8]张发根,傅金贤.浙西南地区华重楼杉木林下栽培技术[J].现代农业科技,2019(14):82,84.[9]谢子芳.杉木林下套种七叶一枝花生长效果研究[J].绿色科技,2017(15):99-101.[10]殷莲华.毛竹林下复合经营七叶一枝花效果分析[J].防护林科技, 2020(12):29-32.[11]龚范武,龚舟,彭静,等.不同林下七叶一枝花栽培技术研究[J].中国农学通报,2015,31(13):155-158.[12]戴兴福.林分类型和郁闭度对林下套种七叶一枝花的影响[J].林业勘察设计,2021(4):56-58.[13]王邦富.不同立地质量对七叶一枝花根系分布及生长的影响[J].中国野生植物资源,2016,35(6):69-71.[14]阮召群.华重楼种植基地土壤肥力综合评价[J].福建农业科技,2016(Z1):1-4.[15]王晓明,秦长光,曾慧杰,等.厚朴林下重楼白粉病防治试验[J].湖南林业科技,2017,44(6):8-11.[16]陈金叶.七叶一枝花不同生长期养分含量及干物质积累特性分析[J].福建农业科技,2022,53(2):32-36.[17]曹建华,陶忠良,蒋菊生,等.不同年龄橡胶树各器官养分含量比较研究[J].热带作物学报,2010,31(8):1317-1323.[18]赵瑞芬,张一弓,张强,等.核桃树体不同器官氮㊁磷㊁钾含量及累积分配特征[J].中国农学通报,2014,30(22):41-44.[19]莫江明,张德强,黄忠良,等.鼎湖山南亚热带常绿阔叶林植物营养元素含量分配格局研究[J].热带亚热带植物学报,2000,8(3):198-206.[20]施家月,王希华,闫恩荣,等.浙江天童常见植物幼树器官的氮磷养分特征[J].华东师范大学学报(自然科学版),2006(2):121-129. [21]祝丽香,王建华,耿慧云,等.桔梗的干物质累积及氮㊁磷㊁钾养分吸收特点[J].植物营养与肥料学报,2010,16(1):197-202.[22]林茂祥,韩凤,刘杰,等.白及氮㊁磷㊁钾养分的吸收与分配[J].中药材, 2017,40(2):253-257.[23]罗佳,陈永忠,周小玲,等.油茶林果实成熟期养分分配特征[J].经济林研究,2017,35(3):102-108.[24]贺金生,韩兴国.生态化学计量学:探索从个体到生态系统的统一化理论[J].植物生态学报,2010,34(1):2-6.[25]ÅGREN G I.Stoichiometry and nutrition of plant growth in natural com-munities[J].Annual review of ecology,evolution,and systematics,2008, 39:153-170.[26]KOERSELMAN W,MEULEMAN A F M.The vegetation NʒP ratio:A new tool to detect the nature of nutrient limitation[J].Journal of applied ecology,1996,33(6):1441-1450.[27]VENTERINK H O,WASSEN M J,VERKROOST A W M,et al.Species richness-productivity patterns differ between N-,P-,and K-limited wet-lands[J].Ecology,2003,84(8):2191-2199.[28]彭玉华,路刚,郝海坤,等.不同种源红锥叶片营养元素分析[J].西南林业大学学报,2012,32(5):37-41.201㊀㊀㊀㊀㊀㊀㊀㊀㊀㊀安徽农业科学㊀㊀㊀㊀㊀㊀㊀㊀㊀㊀㊀㊀㊀㊀㊀㊀㊀㊀㊀㊀㊀㊀㊀㊀㊀2024年。
Au2、Y2和AuY小团簇分子结构与势能函数
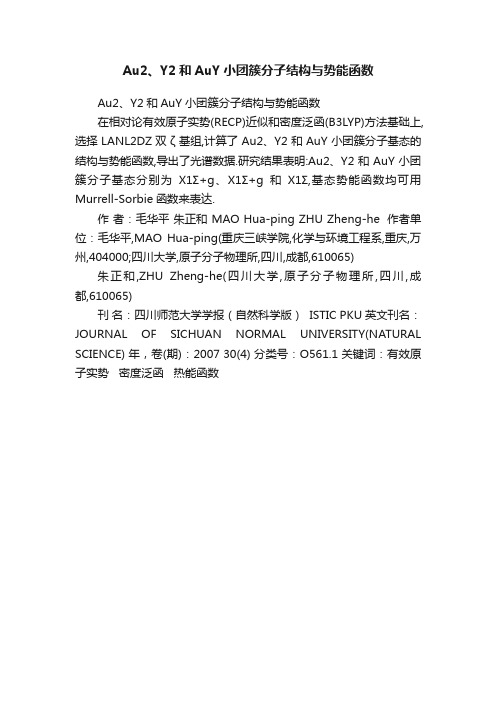
Au2、Y2和AuY小团簇分子结构与势能函数
Au2、Y2和AuY小团簇分子结构与势能函数
在相对论有效原子实势(RECP)近似和密度泛函(B3LYP)方法基础上,选择LANL2DZ双ζ基组,计算了Au2、Y2和AuY小团簇分子基态的结构与势能函数,导出了光谱数据.研究结果表明:Au2、Y2和AuY小团簇分子基态分别为X1Σ+g、X1Σ+g和X1Σ,基态势能函数均可用Murrell-Sorbie函数来表达.
作者:毛华平朱正和 MAO Hua-ping ZHU Zheng-he 作者单位:毛华平,MAO Hua-ping(重庆三峡学院,化学与环境工程系,重庆,万州,404000;四川大学,原子分子物理所,四川,成都,610065)
朱正和,ZHU Zheng-he(四川大学,原子分子物理所,四川,成都,610065)
刊名:四川师范大学学报(自然科学版) ISTIC PKU英文刊名:JOURNAL OF SICHUAN NORMAL UNIVERSITY(NATURAL SCIENCE) 年,卷(期):2007 30(4) 分类号:O561.1 关键词:有效原子实势密度泛函热能函数。
纳米级光滑石墨表面的拉曼光谱表征
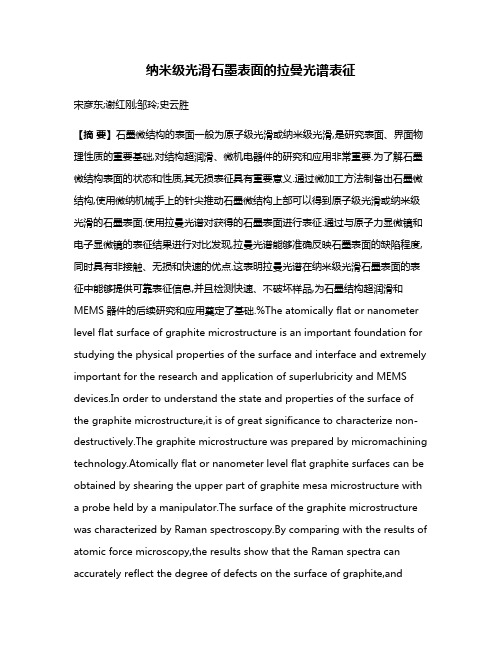
纳米级光滑石墨表面的拉曼光谱表征宋彦东;谢红刚;邹玲;史云胜【摘要】石墨微结构的表面一般为原子级光滑或纳米级光滑,是研究表面、界面物理性质的重要基础,对结构超润滑、微机电器件的研究和应用非常重要.为了解石墨微结构表面的状态和性质,其无损表征具有重要意义.通过微加工方法制备出石墨微结构,使用微纳机械手上的针尖推动石墨微结构上部可以得到原子级光滑或纳米级光滑的石墨表面.使用拉曼光谱对获得的石墨表面进行表征.通过与原子力显微镜和电子显微镜的表征结果进行对比发现,拉曼光谱能够准确反映石墨表面的缺陷程度,同时具有非接触、无损和快速的优点.这表明拉曼光谱在纳米级光滑石墨表面的表征中能够提供可靠表征信息,并且检测快速、不破坏样品,为石墨结构超润滑和MEMS器件的后续研究和应用奠定了基础.%The atomically flat or nanometer level flat surface of graphite microstructure is an important foundation for studying the physical properties of the surface and interface and extremely important for the research and application of superlubricity and MEMS devices.In order to understand the state and properties of the surface of the graphite microstructure,it is of great significance to characterize non-destructively.The graphite microstructure was prepared by micromachining technology.Atomically flat or nanometer level flat graphite surfaces can be obtained by shearing the upper part of graphite mesa microstructure with a probe held by a manipulator.The surface of the graphite microstructure was characterized by Raman spectroscopy.By comparing with the results of atomic force microscopy,the results show that the Raman spectra can accurately reflect the degree of defects on the surface of graphite,andhave the advantages of non-contact,non-destructive and fast.This shows that Raman spectroscopy can provide reliable characterization information in the characterization of the atomically flat graphite surface,and it can be used for rapid and nondestructive testing of samples,which lays the foundation for further research and application of graphite superlubricity and MEMS devices.【期刊名称】《光散射学报》【年(卷),期】2017(029)002【总页数】4页(P138-141)【关键词】拉曼光谱;石墨;纳米级光滑;表征【作者】宋彦东;谢红刚;邹玲;史云胜【作者单位】湖北工业大学电气与电子工程学院,武汉 430068;湖北工业大学电气与电子工程学院,武汉 430068;湖北工业大学电气与电子工程学院,武汉 430068;清华大学精密仪器系,北京 100084;军械工程学院电子与光学工程系,石家庄 050003【正文语种】中文【中图分类】O433.4由于碳原子的排列和成键方式及层状结构,石墨具有很多特殊的物理性质,如较高的熔点、稳定性强而且易导电[1-3]。
- 1、下载文档前请自行甄别文档内容的完整性,平台不提供额外的编辑、内容补充、找答案等附加服务。
- 2、"仅部分预览"的文档,不可在线预览部分如存在完整性等问题,可反馈申请退款(可完整预览的文档不适用该条件!)。
- 3、如文档侵犯您的权益,请联系客服反馈,我们会尽快为您处理(人工客服工作时间:9:00-18:30)。
LETTERdoi:10.1038/nature14295 Square ice in graphene nanocapillariesG.Algara-Siller1,O.Lehtinen1,F.C.W ang2,R.R.Nair3,U.Kaiser1,H.A.Wu2,A.K.Geim3&I.V.Grigorieva3Bulk water exists in many forms,including liquid,vapour and nu-merous crystalline and amorphous phases of ice,with hexagonal ice being responsible for the fascinating variety of snowflakes1,2.Much less noticeable but equally ubiquitous is water adsorbed at interfaces and confined in microscopic pores.Such low-dimensional water de-termines aspects of various phenomena in materials science,geology, biology,tribology and nanotechnology3–8.Theory suggests many pos-sible phases for adsorbed and confined water9–17,but it has proved challenging to assess its crystal structure experimentally17–23.Here we report high-resolution electron microscopy imaging of water locked between two graphene sheets,an archetypal example of hydrophobic confinement.The observations show that the nanoconfined water at room temperature forms‘square ice’—a phase having symmetry qualitatively different from the conventional tetrahedral geometry of hydrogen bonding between water molecules.Square ice has a high packing density with a lattice constant of2.83A˚and can assemble in bilayer and trilayer crystallites.Molecular dynamics simulations indicate that square ice should be present inside hydrophobic nano-channels independently of their exact atomic nature.Many molecular dynamics(MD)studies9–17have explored the struc-ture of low-dimensional water and predicted a great variety of phases, with results sensitive to modelled conditions and sometimes appearing conflicting.For example,buckled monolayer ice10and flat hexagonal ice14,16,23,respectively,were found inside hydrophilic and hydrophobic nanochannels below room temperature,whereas no in-plane order was observed for water inside simulated mica(hydrophilic)and graphite(hy-drophobic)nanochannels at and above room temperature13,15.A close analogue of planar square ice was seen in MD simulations of water inside carbon nanotubes9,11,12,18,where water molecules form a mono-layer that can be viewed as a sheet of square ice rolled up into a quasi-one-dimensional cylinder.Neutron studies18revealed features consistent with the existence of such‘ice nanotubes’,which melted above50K.Two-dimensional(2D)ices have also been found experimentally on the sur-faces of mica and graphite19–23.The studies using scanning probe microscopy20–22and electron crystallography19,23show that near-surface water can form correlated,solid-like layers.As for their intralayer struc-ture,information is only available for2D ices grown below150K,which were found to be hexagonal,with in-plane coordination similar to that of bulk ices19,23.For this study,we deposited a graphene monolayer on a standard trans-mission electron microscopy(TEM)grid,exposed it to a small amount of water and covered it with another graphene monolayer24,25(see‘Sample preparation’in Methods for full details).Most of the water was squeezed out by van der Waals forces that brought the two graphene layers to-gether,but some water remained trapped in numerous pockets of sub-micrometre size(Extended Data Fig.1).We also prepared reference samples similar to those described in ref.24,in which a larger amount of deposited water resulted in three-dimensional droplets of,10–100nm inthickness(ExtendedDataFig.2).TheTEMstudieswere carried outusing transmission electron microscope FEI TITAN80-300operated at80kV. Typical atomic-resolution images of graphene-confined water are shown in Fig.1a and Extended Data Figs1b and3a–i.High-contrast dark spots correspond to oxygen and indicate the positions of the water mole-cules.Hydrogen atoms yield too little contrast to be resolved even by the state-of-the-art TEM.The encapsulating graphene layers are seen in some parts of the images as a faint background with hexagonal sym-metry25,26.The images can easily be improved by digitally subtracting the contribution from the encapsulating graphene25,but we avoid this additional processing in view of the quality and contrast of our raw data, which clearly show water forming a regular square lattice.Fast Fourier transform analysis of the ice lattice yielded a distance a between nearest-neighbour oxygen atoms of2.8360.03A˚(Fig.1a),with no alignment with the graphene lattice observed.We note that the atomic-resolution imaging of interfacial ice is possible only because of the use of graphene: its low atomic number and crystallinity result in a minimal background and high contrast for oxygen atoms24,26,and its mechanical strength,high thermal and high electrical conductivity and chemical stability protect encapsulated water from sublimation and the adverse effects of electron irradiation25,27(see‘Transmission electron microscopy’in Methods). To confirm that the observed square lattice is indeed formed by water, we acquired electron energy loss spectra(EELS)from areas with ice such as shown in Fig.1a and from areas without any visible amount of trapped water.Figure1b compares typical spectra around the oxygen K-edge (which are sensitive to the state of water28).The ice-free areas show little oxygen signal.In contrast,square ice exhibits a spectrum with an overall shape that is qualitatively similar to EELS for three-dimensional ices such as hexagonal I h and diamond cubic I c ices28(inset of Fig.1b). But there are also notable differences,such as the approximately6-eV difference between the main EELS peak positions for square ice and for three-dimensional ices.The energy separation between the main and secondary EELS peaks gives an independent estimate of the oxygen–oxygen distance of about2.8A˚(see‘EELS analysis’in Methods),in agree-ment with the observed lattice constant.Also,EELS measured for large water droplets found in our reference samples agree with the spectra expected for mixtures of liquid water and water vapour(Extended Data Fig.2b).The square ice is found to be highly mobile under the electron beam (Extended Data Fig.3and Supplementary Video),with uniform mono-layers starting to break into crystallites about10nm across with sharp crystallographic edges after the first few seconds of imaging.The crys-tallites change shape,split and merge,with the frequency of reconstruc-tion increasing with beam current.Grain boundaries and dislocations form as the crystallites move and coalesce,but high crystallinity is pre-served with no sign of ice melting or amorphization(Extended Data Fig.3).The strong TEM contrast variations seen in such images arise from differences in ice layer numbers,as illustrated in Fig.2,where equal-height steps in contrast correspond to changes from monolayer to bilayer and to trilayer ice(see Extended Data Fig.4for details).Using the quantified contrast to examine many images and videos of2D ice, we found that three is the maximum number of ice layers observed in our experiments.The TEM images show unambiguously that water molecules in the few-layer ice are AA stacked,with oxygen atoms in adjacent layers sitting directly on top of each other.The AA stacking is evident when1Central Facility for Electron Microscopy,Group of Electron Microscopy of Materials Science,University of Ulm,89081Ulm,Germany.2Chinese Academy of Sciences Key Laboratory of Mechanical Behavior and Design of Materials,Department of Modern Mechanics,University of Science and Technology of China,Hefei,Anhui230027,China.3School of Physics and Astronomy,University of Manchester, Manchester M139PL,UK.26M A R C H2015|V O L519|N A T U R E|443comparing experimental (Fig.2a)with simulated (Fig.2b,c)TEM images:AB stacking results in oxygen atoms in the second layer occu-pying the sites between oxygen sites in the first and third layers,which in turn results in a perceived 45u rotation of the water lattice and reduc-tion in the projected oxygen spacing.Furthermore,the reduced spacing leads to lower contrast and,consequently,a qualitatively different appear-ance of AB bilayers (Fig.2c)compared to the experimental images (Fig.2a).Note that stacking controls the difference between different phases of bulk ice.For example,hexagonal and diamond cubic ices both consist of puckered hexagonal layers but have AB and ABC stacking,respectively.In all bulk ice phases the bonding between water molecules follows so-called ‘ice rules’that require a tetrahedral coordination of hydrogen bonds 1,2.In contrast,the few-layer ice we report here corre-sponds to 90u hydrogen bonding both within and between layers.To support our TEM observations,we carried out MD simulations of water in graphene nanocapillaries (Methods).Distances between thecarbon plane centres were varied from 6.5A˚to 11.5A ˚,to accommodate one to three monolayers of water (Extended Data Fig.5).For narrow 2D channels that can accommodate only one monolayer,we always foundsquare ice with a 52.8160.02A˚,in agreement with experiment (Fig.2d and Extended Data Fig.6).This MD result is robust,showing little dependence on the capillary width,applied pressure or whether graphene sheets were made rigid,flexible or freely moving (Methods).For wider capillaries that accommodate two or three monolayers of water,no in-plane ordering could be found under simulated ambient conditions.Instead,water molecules form a distinct layered structure but locally maintain the tetrahedral arrangement of hydrogen bonds both within and between the layers (Extended Data Fig.7a,b).We believeaIce regionReference regionSimulationb5205405605801 I n t e n s i t y (a r b i t r a r y u n i t s )Energy loss (eV)540560580I n t e n s i t y (a .u .)Energy loss (eV)Figure 1|Square ice.a ,Part of a large water pocket (see Extended Data Fig.1a).Several such pocketswerestudied.Irregular structures are hydrocarbon contamination.The top rightinset showsa magnified image of the area outlined in red.The top left inset shows a Fourier transform of the entire image,with four first-order maxima of the square lattice;the square symmetry ishighlighted by the blue lines;the two hexagonal sets come from encapsulating graphene.b ,EELS from areas containing 2D ice and no visiblewater.Both spectra come from similar size areas (,100nm).The inset shows the simulatedEELS for three-dimensional ices (I c and I h exhibit similar spectra)28.b ca dABFigure 2|Few-layer ice and its stacking order.a ,Isolated crystallite with avarying number of layers.The moire´pattern (seen clearly at the top)is due to encapsulating graphene.The red curve shows changes in the contrast averaged over the corresponding parts of the image (Methods).The changes occur in quantized steps.b and c ,Simulated TEM images for monolayer,bilayer andtrilayer ice with AA and AB stacking,respectively.The AA stacking agrees well with our experimental images whereas the AB stacked ice results in thequalitatively different appearance.d ,Typical snapshot of MD-simulated water in a graphene nanocapillary.Red and white circles show oxygen and hydrogen atoms,respectively;short blue lines indicate hydrogen bonds within H 2O.LETTER444|N A T U R E |V O L 519|26M A R C H 2015this apparent disagreement between simulation and experiment arises because adhesion between the encapsulating graphene sheets imposes pressure on the water as it is squeezed into a small volume,and this needs to be taken into account in simulations.As illustrated in Extended Data Fig.8,this pressure—we refer to it as van der Waals pressure—can be estimated from the energy gain due to such squeezing according to P W<E W/d<1GPa,where E W<20–30meV A˚22is the adhesion en-ergy29,30and d<3.5A˚is a typical interlayer distance.This estimate agrees with our simulations of hydrostatic pressure acting on a gas trapped between freely moving graphene sheets(Methods).To mimic the effect of van der Waals pressure,we ran simulations with an applied external pressure P(as illustrated in Extended Data Fig.5)and found a pro-nounced transition from in-plane disorder at low P to layered ice at P.1GPa(Extended Data Figs7and9).The layered ice exhibited the same lattice constant(a52.8160.02A˚)as the monolayer,in agree-ment with experiment.The MD simulations fail,however,in reproducing the observed AA stacking and instead show a tendency for crystals with AB stacking or no interlayer order(Extended Data Figs7and9).The disagreement is perhaps not surprising when we consider that as P increases to reach the crystallization transition,hydrogen bonds switch to the in-plane con-figuration(Extended Data Figs7d and9b)so that coupling between monolayers of square ice becomes van-der-Waals-like.Such weak cou-pling is known to be extremely difficult to accurately account for theo-retically.Moreover,in the experiment the graphene-confined ice exhibits sharp steps between terraces(see Fig.2),which can result in extra lateral forces acting on different layers not considered in the simulations. Finally,our simulations for bilayer and trilayer ice yield an interlayer separation of c52.860.3A˚,which gives a crystal lattice close to the simple cubic structure(c5a)and a density approximately1.5times higher than that of common ice.But the exact structure is still,strictly speaking,tetragonal because of the qualitative difference between inter-layer and intralayer bonding(Extended Data Figs7and9).The2D ice we report here exhibits coordination that is qualitatively different from the conventional tetrahedral coordination in bulk and sur-face ices.We expect it to be common inside hydrophobic nanocapillaries under ambient conditions,basically because the water–surface interaction in such confinement is much weaker than the interaction between water molecules.Indeed,our MD simulations yield the same square lattices inside non-graphene capillaries with widely varying hydrophobicity (Extended Data Fig.10).The existence of low-dimensional ice at room temperature has been proposed to explain the fast water permeation through hydrophobic nanocapillaries,including carbon nanotubes and graphene-based membranes,and our report supports this idea.The van der Waals pressure invoked here may be important in many nano-scale phenomena.Online Content Methods,along with any additional Extended Data display items and Source Data,are available in the online version of the paper;references unique to these sections appear only in the online paper.Received10October2014;accepted4February2015.1.Petrenko,V.F.&Whitworth,R.W.Physics of Ice(Oxford Univ.Press,2002).2.Malenkov,G.Liquid water and ices:understanding the structure and physicalproperties.J.Phys.Condens.Matter21,283101(2009).3.Israelachvili,J.&Wennerstro¨m,H.Role of hydration and water structure inbiological and colloidal interactions.Nature379,219–225(1996).4.Brown,G.E.How minerals react with water.Science294,67–69(2001).5.Appelo,C.A.J.&Postma,D.Geochemistry,Groundwater and Pollution(Taylor&Francis,2005).6.Chandler,D.Interfaces and the driving force of hydrophobic assembly.Nature437,640–647(2005).7.Verdaguer,A.,Sacha,G.M.,Bluhm,H.&Salmeron,M.Molecular structure of waterat interfaces:wetting at the nanometer scale.Chem.Rev.106,1478–1510(2006).8.Chaplin,M.F.in Adsorption and Phase Behaviour in Nanochannels and Nanotubes(eds Dunne L.&Manos,G.)241–255(Springer,2010).9.Kalra,A.,Garde,S.&Hummer,G.Osmotic water transport through carbonnanotube membranes.Proc.Natl A100,10175–10180(2003).10.Zangi,R.&Mark,A.E.Monolayer ice.Phys.Rev.Lett.91,025502(2003).11.Maniwa,Y.et al.Ordered water inside carbon nanotubes:formation of pentagonalto octagonal ice-nanotubes.Chem.Phys.Lett.401,534–538(2005).12.Takaiwa,D.,Hatano,I.,Koga,K.&Tanaka,H.Phase diagram of water in carbonnanotubes.Proc.Natl A105,39–43(2008).13.Giovambattista,N.,Rossky,P.J.&Debenedetti,P.G.Phase transitions induced bynanoconfinement in liquid water.Phys.Rev.Lett.102,050603(2009).14.Han,S.,Choi,M.Y.,Kumar,P.&Stanley,H.E.Phase transitions in confined waternanofilms.Nature Phys.6,685–689(2010).15.Srivastava,R.,Docherty,H.,Singh,J.K.&Cummings,P.T.Phase transitions ofwater in graphite and mica pores.J.Phys.Chem.C115,12448–12457(2011).16.Bai,J.&Cheng Zeng,X.Polymorphism and polyamorphism in bilayer waterconfined to slit nanopore under high pressure.Proc.Natl A109,21240–21245(2012).17.Nair,R.R.,Wu,H.A.,Jayaram,P.N.,Grigorieva,I.V.&Geim,A.K.Unimpededpermeation of water through helium-leak–tight graphene-based membranes.Science335,442–444(2012).18.Kolesnikov,A.I.et al.Anomalously soft dynamics of water in a nanotube:arevelation of nanoscale confinement.Phys.Rev.Lett.93,035503(2004).19.Yang,D.S.&Zewail,A.H.Ordered water structure at hydrophobic graphiteinterfaces observed by4D,ultrafast electron crystallography.Proc.Natl Acad.Sci.USA106,4122–4126(2009).20.Xu,K.,Cao,P.&Heath,J.R.Graphene visualizes the first water adlayers on mica atambient conditions.Science329,1188–1191(2010).21.He,K.T.,Wood,J.D.,Doidge,G.P.,Pop,E.&Lyding,J.W.Scanning tunnellingmicroscopy study and nanomanipulation of graphene-coated water on mica.Nano Lett.12,2665–2672(2012).22.Zheng,Y.,Su,C.,Lu,J.&Loh,K.P.Room-temperature ice growth on graphiteseeded by nano-graphene oxide.Angew.Chem.Int.Ed.52,8708–8712(2013).23.Kimmel,G.A.et al.No confinement needed:observation of a metastablehydrophobic wetting two-layer ice on graphene.J.Am.Chem.Soc.131,12838–12844(2009).24.Yuk,J.M.et al.High-resolution EM of colloidal nanocrystal growth using grapheneliquid cells.Science336,61–64(2012).25.Algara-Siller,G.,Kurasch,S.,Sedighi,M.,Lehtinen,O.&Kaiser,U.The pristineatomic structure of MoS2monolayer protected from electron radiation damage by graphene.Appl.Phys.Lett.103,203107(2013).26.Meyer,J.C.,Girit,C.O.,Crommie,M.F.&Zettl,A.Imaging and dynamics of lightatoms and molecules on graphene.Nature454,319–322(2008).27.Dubochet,J.,Lepault,J.,Freeman,R.,Berriman,J.A.&Homo,J.-C.Electronmicroscopy of frozen water and aqueous solutions.J.Microsc.128,219–237 (1982).28.Kobayashi,K.,Koshino,M.&Suenaga,K.Atomically resolved images of I h ice singlecrystals in the solid phase.Phys.Rev.Lett.106,206101(2011).29.Bjo¨rkman,T.,Gulans,A.,Krasheninnikov,A.V.&Nieminen,R.M.Van der Waalsbonding in layered compounds from advanced density-functional first-principles calculations.Phys.Rev.Lett.108,235502(2012).30.Koenig,S.P.,Boddeti,N.G.,Dunn,M.L.&Bunch,J.S.Ultrastrong adhesion ofgraphene membranes.Nature Nanotechnol.6,543–546(2011). Supplementary Information is available in the online version of the paper. Acknowledgements This work was supported by the DFG(Germany),the European Research Council,the EU Graphene Flagship,the National Natural Science Foundation of China,the Ministry of Science,Research and Arts of Baden-Wuerttemberg (Germany),the Office of Naval Research,the Air Force Office of Scientific Research,the Anhui Provincial Natural Science Foundation(China),the Finnish Cultural Foundation and the Fundamental Research Funds for the Central Universities of China.MD simulations were carried out at Supercomputing Center of the University of Science and Technology of China.Author Contributions U.K.,I.V.G and A.K.G.proposed and directed the project.G.A.-S. and O.L.designed the experiments and samples,performed TEM measurements and analysed them.H.A.W.and F.C.W.carried out MD simulations(with feedback from A.K.G.).I.V.G.and A.K.G.wrote the manuscript with help from all the authors.Author Information Reprints and permissions information is available at/reprints.The authors declare no competing financial interests. Readers are welcome to comment on the online version of the paper. Correspondence and requests for materials should be addressed toI.V.G.(irina.grigorieva@),U.K.(ute.kaiser@uni-ulm.de)orH.A.W.(wuha@).LETTER26M A R C H2015|V O L519|N A T U R E|445METHODSSample preparation.Graphene monolayers were grown on Cu foils by chemical vapour deposition and then transferred onto gold Quantifoil grids(Au mesh cov-ered with an amorphous carbon film having a dense array of holes with a diameter of1.2m m).Importantly,a thin layer of Pt was deposited on the TEM grids before graphene transfer in order to reduce hydrocarbon contamination31.For the trans-fer,several Quantifoil grids were immersed in isopropanol and then placed‘face down’onto a piece of graphene on copper about1cm2in size.As the isopropanol evaporated,the amorphous carbon film of the TEM grid came into contact with graphene and became attached to it32.After that the assembly was floated on the surface of ammonium peroxodisulfate for several hours until all copper was slowly etched away,leaving free-floating graphene with several TEM grids attached to it. This was broken into pieces,with one grid attached to each,washed in water and isopropanol and left to dry in air at room temperature.After that1m l of deionized water was carefully cast on top of one of the grids,and another graphene-covered grid was placed on top,covering the water droplet.The resulting sandwich was left to dry under ambient conditions overnight,during which time the water drop slowly evaporated,bringing the two graphene layers together and gradually squeezing the liquid out so that only a small amount of adsorbed water remained captured in between the graphene sheets.TEM.The use of both a relatively low operating voltage(80keV)and the aberra-tion correction were essential in our study:higher voltages are known to produce knock-on damage in graphene33and the aberration correction,even at relatively low acceleration voltages,allows atomic-resolution imaging of both graphene and water.The spherical aberration coefficient was set to30m m and the sample was imaged at Scherzer focus,resulting in dark atom contrast.The background pres-sure in the microscope was,1028mbar,and all experiments were carried out at room temperature.Aberration-corrected high-resolution TEM images were acquired at a dose of33104electrons per nm2and an exposure time of,1s per frame.The exposure of the studied area before acquisition of the reported images was approxi-mately600electrons per nm2.No notable heating by the electron beam is expected under these operating conditions.Indeed,for amorphous carbon films under sim-ilar experimental conditions,the heating was estimated to be,1K(ref.34).As ther-mal conductivity of graphene is3,000times higher than that of amorphous carbon, we expect even less heating in our case.This agrees with our observation that the appearance of square ice did not qualitatively change with increasing or decreasing of the beam current.The majority of our samples remained intact during the relatively long expo-sures to the electron beam(typically,10–20min);in a few cases only,we noticed etching of graphene27,33–36.This is in contrast to the earlier work on frozen aqueous samples that suffered from fast sublimation under the electron beam27,28,37.The high stability of2D ice is due to graphene encapsulation.The highly conductive gra-phene layers(both electrically and thermally)provide an efficient channel for ab-sorbing and rapidly dissipating the energy introduced by electron impacts,thus reducing the damage to ice crystals.This effect of graphene encapsulation was re-cently demonstrated in TEM studies25,38of monolayer MoS2,a radiation-sensitive material.The encapsulation reduced the damage rate by nearly three orders of magnitude as compared to non-encapsulated MoS2.Similarly,graphene capillaries in the present work served not only as a confinement channel but also as protection against the beam damage,which enabled us to observe the ice crystals without immediately evaporating or destroying them.EELS analysis.Electron energy loss spectra39,40were acquired using GIF Quantum ER filter in the diffraction mode(convergence angle of1.8mrad and collection angle of4.4mrad).The spectra were recorded with exposure and integration times of5s and200s,respectively.A low pass filter(two pixels)and linear background sub-traction were applied.All EELS showed prominent signals from carbon and oxy-gen.Hydrogen is not detectable with the instrument.No other elements could be detected,except for a small amount of Si that is commonly present in all areas because of contamination during sample preparation41.The overall appearance of EELS curves allows us to distinguish immediately be-tween ice and mixtures of liquid water and water vapour(compare Figure1b and Extended Data Fig.2).Furthermore,bulk ices such as I c and I h have rather similar spectra28,more similar to each other than to the observed EELS for square ice.This is understandable given that both I c and I h consist of puckered hexagonal layers,which is different from the planar square configuration.We have also used the spectral positions of the main and secondary peaks(see Fig.1b)to estimate interatomic dis-tances in the square ice.Secondary peaks(after the main ionization threshold that happens in our case at about540eV;Fig.1b)arise owing to multiple scattering,and the energy separation D E obeys the relation42D E3R25C,where R is the distance between oxygen atoms and C is a ing C5150(this value was empir-ically determined for cubic ice43,which is the closest approximation to square ice to be found in the literature)and an energy of about559eV for the prominent sec-ondary peak in the observed EELS,we estimate R52.8060.07A˚.The good agreement between this value and a obtained from atomic-resolution TEM images provides an independent confirmation of the crystal structure of square ice.In principle,the interlayer separation in layered crystals can be determined by TEM imaging at different tilt angles.Unfortunately,this is impossible to achieve in practice for our nanoscale crystals,which constantly move and rotate under the electron beam.Indeed,for acquiring interpretable high-resolution images,crystals need to be oriented precisely along a zone axis and remain stationary during im-aging.The reason that high-resolution imaging is possible in our case is that the graphene layers fix the orientation of ice crystals relative to the electron beam.Even though the crystals change their in-plane orientation(rotate),the[001]direction remains parallel to the optical axis of the microscope.Analysis of TEM images.To determine the number of layers in2D ice crystallites, we quantified the contrast from oxygen atoms using a variance filter,as illustrated step by step in Extended Data Fig.4and explained in its legend.This resulted in contrast maps such as that in Extended Data Fig.4d.They show that the average contrast changes in steps of equal height.There are three distinct parts in the ice crystallite in Extended Data Fig.4a,of one,two and three monolayers of water each. The simulated images presented in Fig.2b and c were obtained as follows.First, we constructed a square lattice of water molecules arranged in one,two or three layers with different stacking orders.After that,TEM images for different arrange-ments were simulated using QSTEM software44and the parameters correspond-ing to our experimental conditions(accelerating voltage and spherical aberration coefficient).MD setup.Most of our simulations were done for the geometry shown in Extended Data Fig.5.This involved two water reservoirs that contained2,000molecules each and were connected by a relatively long capillary formed by two parallel gra-phene sheets.The length and width of the graphene channel were kept at68A˚and 56A˚,respectively.The height h was chosen to be6.5A˚,9.0A˚and11.5A˚in order to accommodate one,two and three layers of water molecules,respectively.The gra-phene sheets were either kept rigid or allowed to be flexible during simulations. Unless stated otherwise,water was modelled using the extended simple point charge(SPC/E)model,which is described by the sum of a long-range Coulomb potential and a short-range Lennard–Jones potential45.The parameters for water–graphene interaction were chosen as in ref.46.The long-range interactions were computed using the particle–particle particle–mesh(PPPM)algorithm,with a con-vergence parameter of1024.Periodic boundary conditions were applied in all three directions of the simulation box.For monolayer ice,we have also performed MD simulations for freely moving graphene sheets that‘self-consistently’enclose trapped water molecules(Extended Data Fig.8d).For bilayer and trilayer ice,the graphene confinement observed experimentally is terraced with atomically sharp steps between ice terraces(see Fig.2).It has proved difficult to reproduce such a terraced confinement in MD ana-lysis.Therefore,the high pressure induced by encapsulating graphene sheets(see below)was modelled to a first approximation by applying a hydrostatic pressure P in the direction parallel to the graphene layers,as shown in Extended Data Fig.5. MD simulations were performed in an isothermal,isobaric ensemble,in which temperature(298K)and pressure were controlled by the Nose–Hoover thermo-stat and barostat,respectively.In the equilibrium run,pressure P was kept at1atm (1024GPa)for5ns,during which time water molecules filled the graphene nano-capillary.After that,P was increased up to10GPa over15ns.A time step of1.0fs was used for the velocity-Verlet integrator.All the simulations were carried out using LAMMPS47.To determine the lattice parameter a,we counted the number of water molecules over the entire area of the graphene capillary.Van der Waals pressure.Attractive van der Waals forces between two graphene sheets favour the adhesion of the sheets over a maximal area.If a material(for ex-ample,a gas bubble)is trapped between the sheets,the bubble will continue to shrink in size until a built-up hydrostatic pressure is able to balance the adhesion forces.For a quasi-2D confinement such as that shown in Extended Data Fig.8a,it is straight-forward to estimate the resulting van der Waals pressure,P W.Indeed,a displace-ment d of the enclosure boundary results in a gain in adhesion energy equal to d3L3E W,where L is the enclosure circumference and E W is the difference be-tween graphene–graphene and graphene–water adhesion energies per unit area.E W can be estimated as a typical value of the adhesion energy for van der Waals materials29,30because the water–graphene interaction is hydrophobic and relatively small.The above displacement requires a work of F3d against the internal pressure P W,where F5P W3d3L.For a monolayer of ice,we can use d<3.5A˚,a typical interlayer distance in van der Waals materials.The equilibrium requires P W5E W/ d,which yields,1GPa for E W<30meV A˚22,found experimentally for mono-layer graphene30.To support this estimate,we have‘measured’the van der Waals pressure in MD simulations.To this end,a small amount of helium gas(1,000atoms)was confined between freely moving graphene sheets and the system was allowed to reach equi-librium(Extended Data Fig.8b,c).The hydrostatic pressure was estimated byLETTER。