Effects of elemental Sn on the properties and inclusions of the free-cutting steel
Effect of the material-hardening mode on the springback
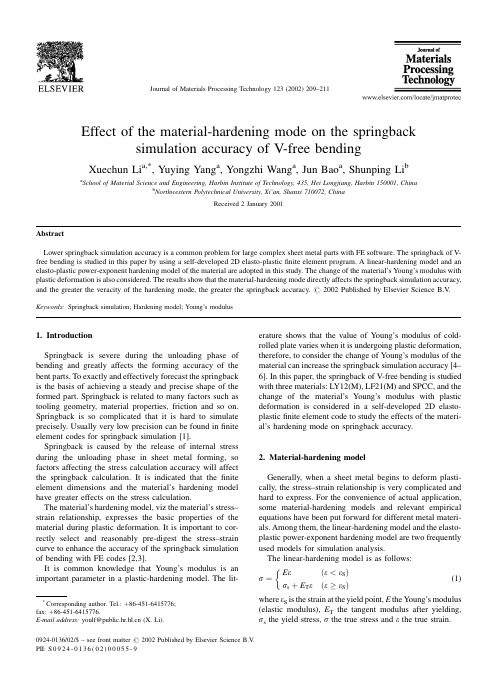
Effect of the material-hardening mode on the springbacksimulation accuracy of V-free bendingXuechun Li a,*,Yuying Yang a ,Yongzhi Wang a ,Jun Bao a ,Shunping Li baSchool of Material Science and Engineering,Harbin Institute of Technology,435,Hei Longjiang,Harbin 150001,ChinabNorthwestern Polytechnical University,Xi’an,Shanxi 710072,ChinaReceived 2January 2001AbstractLower springback simulation accuracy is a common problem for large complex sheet metal parts with FE software.The springback of V-free bending is studied in this paper by using a self-developed 2D elasto-plastic finite element program.A linear-hardening model and an elasto-plastic power-exponent hardening model of the material are adopted in this study.The change of the material’s Young’s modulus with plastic deformation is also considered.The results show that the material-hardening mode directly affects the springback simulation accuracy,and the greater the veracity of the hardening mode,the greater the springback accuracy.#2002Published by Elsevier Science B.V .Keywords:Springback simulation;Hardening model;Young’s modulus1.IntroductionSpringback is severe during the unloading phase of bending and greatly affects the forming accuracy of the bent parts.To exactly and effectively forecast the springback is the basis of achieving a steady and precise shape of the formed part.Springback is related to many factors such as tooling geometry,material properties,friction and so on.Springback is so complicated that it is hard to simulate ually very low precision can be found in finite element codes for springback simulation [1].Springback is caused by the release of internal stress during the unloading phase in sheet metal forming,so factors affecting the stress calculation accuracy will affect the springback calculation.It is indicated that the finite element dimensions and the material’s hardening model have greater effects on the stress calculation.The material’s hardening model,viz the material’s stress–strain relationship,expresses the basic properties of the material during plastic deformation.It is important to cor-rectly select and reasonably pre-digest the stress–strain curve to enhance the accuracy of the springback simulation of bending with FE codes [2,3].It is common knowledge that Young’s modulus is an important parameter in a plastic-hardening model.The lit-erature shows that the value of Young’s modulus of cold-rolled plate varies when it is undergoing plastic deformation,therefore,to consider the change of Young’s modulus of the material can increase the springback simulation accuracy [4–6].In this paper,the springback of V-free bending is studied with three materials:LY12(M),LF21(M)and SPCC,and the change of the material’s Young’s modulus with plastic deformation is considered in a self-developed 2D elasto-plastic finite element code to study the effects of the materi-al’s hardening mode on springback accuracy.2.Material-hardening modelGenerally,when a sheet metal begins to deform plasti-cally,the stress–strain relationship is very complicated and hard to express.For the convenience of actual application,some material-hardening models and relevant empirical equations have been put forward for different metal materi-als.Among them,the linear-hardening model and the elasto-plastic power-exponent hardening model are two frequently used models for simulation analysis.The linear-hardening model is as follows:s ¼E e ðe <e S Þs s þE T e ðe !e S Þ (1)where e S is the strain at the yield point,E the Young’s modulus (elastic modulus),E T the tangent modulus after yielding,s s the yield stress,s the true stress and e the truestrain.Journal of Materials Processing Technology 123(2002)209–211*Corresponding author.Tel.:þ86-451-6415776;fax:þ86-451-6415776.E-mail address:youlf@ (X.Li).0924-0136/02/$–see front matter #2002Published by Elsevier Science B.V .PII:S 0924-0136(02)00055-9The elasto-plastic power-exponent hardening model has the form:s ¼E e ðe <e S ÞK e n ðe !e S Þ(2)where K and n represents the hardening coef ficient and the hardening exponent,respectively.The change of Young ’s modulus with plastic deforming can be expressed as [7]:E ¼E 0ð1þe p Þmwhere E 0is the initial Young ’s modulus and e p the equivalent plastic strain.Through the tensile test,the basic property parameters for three materials:LY12(M),LF21(M)and SPCC,were obtained,as shown in Table 1.And the Young ’s modulus –plastic strain relationships for the materials are shown in Fig.1.3.FEM for the springback simulation of V-free bending The static implicit algorithm is adopted in the FE code to simulate the V-free bending process.The counter node contact force at the end of forming phase is used as the initial acting force for springback calculation.Then an iteration algorithm begins until all the node contact forces becomes 0.The tooling dimensions for V-free bending are shown in Fig.2.In the developed FE code,an elasto-plastic algorithm is used in the loading phase and an elastic algo-rithm in springback,and the four nodes isoparametric plane element is adopted.Also,the change of Young ’s modulus with plastic deformation is considered.4.Results and analysisIn V-free bending simulation,different hardening modes result in different stress fields at the end of bending forming,viz the initial acting force for springback calculation will be different.In the simulation,the material ’s hardening mod-ulus H 0must be calculated to form a constitutive matrix,which can be expressed as:H 0¼d s d e p(3)or H 0¼EE T E ÀE T(4)where E T is the slope of the stress –strain curve after yielding,namely E T ¼d s d e(5)Table 1Basic property parameters Materials s (MPa)E 0(GPa)E T (MPa)K (MPa)n mLY12(M)91.4170.089386.668356.2250.206À0.0855LF21(M)60.0067.339148.15164.1170.183À0.1068SPCC 246.05159.048553.339551.6470.226À0.0351Fig.1.The change of Young ’s modulus with plasticdeformation.Fig.2.Tooling diagram:(1)punch;(2)blank;(3)die.Fig.3.Bending angle vs.springback angle for three materials without considering the change of Young ’s modulus with plastic deformation.BISO —linear-hardening model,MISO —elasto-plastic power-exponent hardening model.210X.Li et al./Journal of Materials Processing Technology 123(2002)209–211It is obvious that the stress obtained with the elasto-plastic power-exponent hardening model is more accurate than that with the linear-hardening model.As a result,the initial acting force for springback calculation is more accurate with the use of the elasto-plastic power-exponent hardening model.Fig.3is the relationship between the bending angle in the forming phase and the springback angle in the springback phase under the two material-hardening models.The bend-ing angle is the supplementary angle of the bent part ’s included angle.The figure shows that the springback calcu-lated with the elasto-plastic power-exponent hardening model is closer to the experiment results than that with the linear-hardening model.Figs.4–6show the relationships between the bending angle in the forming phase and the springback angle in the springback phase for the three materials,with the elasto-plastic power-exponent hardening model including the change of Young ’s modulus with plastic deformation.It has been proven by the experimental results that the value of Young ’s modulus changes along with plastic deformation,so considering the change of Young ’s modulus in the FE code can describe the deforming state of the material more truly,and the springback calculated is closer to the experiment value.5.Conclusions1.The material ’s hardening model directly affects the accuracy of springback calculation.The greater the veracity of the hardening model,the greater the springback accuracy.The springback calculated with the elasto-plastic power-exponent hardening model agrees better with experimental results than that calculated with the linear-hardening model.2.Young ’s modulus has a great effect on springback simulation ing the change of Young ’s modulus with plastic deformation can enhance the accuracy of springback simulation.References[1] D.B.Zhu,Newest progress on the springback ’s study of plate forming,J.Plas.Eng.1(2000)11–17.[2]Z.T.Zhang,D.Lee,Development of a new model for plane strainbending and springback analysis,J.Mater.Eng.Perform.4(3)(1995)291–300.[3]Z.T.Zhang,S.J.Hu,Stress and residual stress distributions in planestrain bending,Int.J.Mech.Sci.40(6)(1998)533–543.[4] A.Makinouchi,H.Ogawa,Use the ITAS-2D Program to Calculate theSpringback with Considering the Change in Young ’s Modulus due to Plastic Deformation,Unite Report Conference of Plastic Deformation,No.43,Tokyo,1992,pp.755–756.[5]L.J.Devin,A.H.Streppl,A process model for air bending,J.Mater.Process.Technol.57(1996)48–54.[6]S.Shima,M.Yang,A study of accuracy in an intelligent V-bendingprocess for sheet metals,Material 44(500)(1995)578–583.[7]X.C.Li,Y .Y .Yang,Discuss on the relationship between the Young ’smodulus and plastic deformation,J.Harbin Inst.Technol.32(5)(2000)54–56.Fig.4.Bending angle vs.springback angle for materialSPCC.Fig.5.Bending angle vs.springback angle for materialLY12(M).Fig.6.Bending angle vs.springback angle for material LF21(M).X.Li et al./Journal of Materials Processing Technology 123(2002)209–211211。
Effect of Nb addition on the microstructure and properties of AlCoCrFeNi high-entropyalloy

article info
Article history: Received 18 January 2011 Received in revised form 20 September 2011 Accepted 28 October 2011 Available online 11 November 2011
Keywords: High entropy alloy Eutectic Laves phase Solid solution
abstract
The microstructures and properties of the AlCoCrFeNbxNi high-entropy alloys (HEAs) were investigated. Two phases were found in the prepared AlCoCrFeNbxNi HEAs: one is body-centered-cubic (BCC) solid solution phase; the other is the Laves phase of (CoCr)Nb type. The microstructures of the alloy series vary from hypoeutectic to hypereutectic, and the compressive yield strength and Vickers hardness have an approximately linear increase with increasing Nb content. The residual magnetization (Mr) reaches a maximum for AlCoCrFeNb0.1Ni alloy, which is 6.106 emu/g. The factor of ˝, which is defined as entropy of mixing times 1000 over enthalpy of mixing, well predicts the phase formation for the multicomponents alloys.
应用地球化学元素丰度数据手册-原版
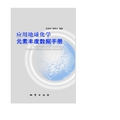
应用地球化学元素丰度数据手册迟清华鄢明才编著地质出版社·北京·1内容提要本书汇编了国内外不同研究者提出的火成岩、沉积岩、变质岩、土壤、水系沉积物、泛滥平原沉积物、浅海沉积物和大陆地壳的化学组成与元素丰度,同时列出了勘查地球化学和环境地球化学研究中常用的中国主要地球化学标准物质的标准值,所提供内容均为地球化学工作者所必须了解的各种重要地质介质的地球化学基础数据。
本书供从事地球化学、岩石学、勘查地球化学、生态环境与农业地球化学、地质样品分析测试、矿产勘查、基础地质等领域的研究者阅读,也可供地球科学其它领域的研究者使用。
图书在版编目(CIP)数据应用地球化学元素丰度数据手册/迟清华,鄢明才编著. -北京:地质出版社,2007.12ISBN 978-7-116-05536-0Ⅰ. 应… Ⅱ. ①迟…②鄢…Ⅲ. 地球化学丰度-化学元素-数据-手册Ⅳ. P595-62中国版本图书馆CIP数据核字(2007)第185917号责任编辑:王永奉陈军中责任校对:李玫出版发行:地质出版社社址邮编:北京市海淀区学院路31号,100083电话:(010)82324508(邮购部)网址:电子邮箱:zbs@传真:(010)82310759印刷:北京地大彩印厂开本:889mm×1194mm 1/16印张:10.25字数:260千字印数:1-3000册版次:2007年12月北京第1版•第1次印刷定价:28.00元书号:ISBN 978-7-116-05536-0(如对本书有建议或意见,敬请致电本社;如本社有印装问题,本社负责调换)2关于应用地球化学元素丰度数据手册(代序)地球化学元素丰度数据,即地壳五个圈内多种元素在各种介质、各种尺度内含量的统计数据。
它是应用地球化学研究解决资源与环境问题上重要的资料。
将这些数据资料汇编在一起将使研究人员节省不少查找文献的劳动与时间。
这本小册子就是按照这样的想法编汇的。
the tyndall effect thus implies

the tyndall effect thus implies“The Tyndall Effect”is a phenomenon often observed in everyday life, in which the scattering of light by suspended particles in a medium leads to the appearance of a visible beam of light. In this article, we will explore the underlying principles behind the Tyndall Effect and delve into its implications in various fields.Firstly, let us understand the basic concept of the Tyndall Effect. Named after the 19th-century physicist John Tyndall, this effect occurs when light encounters particles within a medium, causing some of the light rays to scatter in different directions. The scattered light is then reflected or refracted, creating a visible beam or cone of light. This phenomenon is most noticeable when a beam of light passes through a cloudy liquid or a dusty room, where suspended particles are abundant.To comprehend why the Tyndall Effect occurs, we must delve into the behavior of light waves. Light is composed of electromagnetic waves, which consist of alternating electric and magnetic fields. When light interacts with particles in a medium, such as smoke particles or water droplets, the electric and magnetic fields can induce a dipole moment within the particles. As a result of thisinteraction, the light waves are scattered in various directions.The intensity and color of the scattered light depend on the size of the particles and the wavelength of light. If the particles are larger than the wavelength of incident light, the scattered light will contain various colors, resulting in white light. However, if the particles are smaller than the wavelength of light, the scattering will be more pronounced for shorter wavelengths, such as blue and violet light. This explains why the scattered light appears blue, while the transmitted light through the medium appears yellow or red, as blue light is scattered more strongly in the atmosphere.Now that we have grasped the fundamental principles of the Tyndall Effect, let us explore its implications in various fields. One significant area where the Tyndall Effect is commonly observed is in atmospheric science. This phenomenon plays a crucial role in the scattering of sunlight in the Earth's atmosphere, giving rise to the blue color of the sky. As sunlight encounters tiny molecules and particles in the atmosphere, the shorter blue and violet wavelengths of light are scattered more efficiently, creating the appearance of a blue sky.Additionally, the Tyndall Effect has significant applications in the field of medical diagnostics. This effect is often exploited in technologies such as turbidimetry and nephelometry, which measure the concentration of suspended particles in a liquid sample. By analyzing the scattered light, these techniques allow healthcare professionals to identify abnormalities or monitor the progress of certain diseases, such as kidney disorders or bacterial infections.Furthermore, the Tyndall Effect has numerous applications in industrial processes. For instance, in the field of cosmetics, manufacturers use this phenomenon to create shimmering or sparkling effects in products. By incorporating finely suspended particles that scatter light, such as mica or titanium dioxide, cosmetics can enhance the perceived appearance of skin or add an iridescent quality to lipsticks or nail polishes.In conclusion, the Tyndall Effect is a fascinating phenomenon that arises from the scattering of light by suspended particles in a medium. This effect has implications in various fields, ranging from atmospheric science to medical diagnostics and industrialapplications. By understanding the underlying principles behind the Tyndall Effect, we can appreciate the beauty of everyday occurrences and harness its potential in diverse areas of research and development.。
The Effects of Radiation on Materials Properties
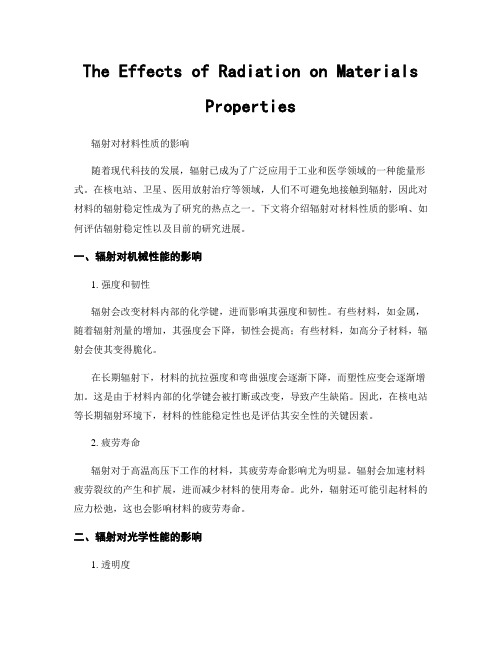
The Effects of Radiation on MaterialsProperties辐射对材料性质的影响随着现代科技的发展,辐射已成为了广泛应用于工业和医学领域的一种能量形式。
在核电站、卫星、医用放射治疗等领域,人们不可避免地接触到辐射,因此对材料的辐射稳定性成为了研究的热点之一。
下文将介绍辐射对材料性质的影响、如何评估辐射稳定性以及目前的研究进展。
一、辐射对机械性能的影响1. 强度和韧性辐射会改变材料内部的化学键,进而影响其强度和韧性。
有些材料,如金属,随着辐射剂量的增加,其强度会下降,韧性会提高;有些材料,如高分子材料,辐射会使其变得脆化。
在长期辐射下,材料的抗拉强度和弯曲强度会逐渐下降,而塑性应变会逐渐增加。
这是由于材料内部的化学键会被打断或改变,导致产生缺陷。
因此,在核电站等长期辐射环境下,材料的性能稳定性也是评估其安全性的关键因素。
2. 疲劳寿命辐射对于高温高压下工作的材料,其疲劳寿命影响尤为明显。
辐射会加速材料疲劳裂纹的产生和扩展,进而减少材料的使用寿命。
此外,辐射还可能引起材料的应力松弛,这也会影响材料的疲劳寿命。
二、辐射对光学性能的影响1. 透明度辐射对材料的透明度影响尤为明显。
透明材料如石英晶体、玻璃等,辐射会导致其透明度下降,进而影响材料的使用寿命和性能。
此外,辐射还会引起材料的荧光或辐射缺陷,也会影响材料的光学性能。
2. 抗辐射性对于强辐射环境下工作的光学元件,其抗辐射性尤为重要。
辐射剂量越大,光学元件的辐射损伤就越严重。
因此,在设计强辐射环境下的光学系统时,要充分考虑光学元件的抗辐射性。
三、如何评估材料的辐射稳定性目前,对于材料的辐射评估主要采用以下方法:1. 辐射损伤试验这种方法主要是通过模拟实际辐射环境下的材料应力状态和辐射剂量,来评估材料在长期辐射下的稳定性。
常见的辐射损伤试验有离子束注入、中子辐射、质子辐射等。
2. 吸收剂量吸收剂量是衡量辐射对材料影响的一个重要指标。
nb微合金化对钢的影响英文
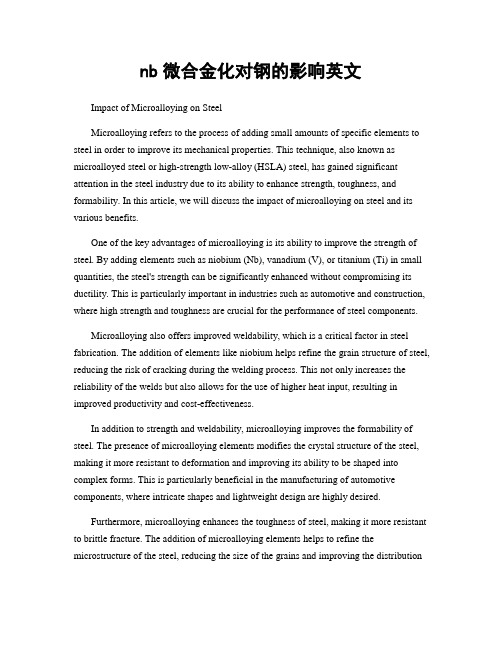
nb微合金化对钢的影响英文Impact of Microalloying on SteelMicroalloying refers to the process of adding small amounts of specific elements to steel in order to improve its mechanical properties. This technique, also known as microalloyed steel or high-strength low-alloy (HSLA) steel, has gained significant attention in the steel industry due to its ability to enhance strength, toughness, and formability. In this article, we will discuss the impact of microalloying on steel and its various benefits.One of the key advantages of microalloying is its ability to improve the strength of steel. By adding elements such as niobium (Nb), vanadium (V), or titanium (Ti) in small quantities, the steel's strength can be significantly enhanced without compromising its ductility. This is particularly important in industries such as automotive and construction, where high strength and toughness are crucial for the performance of steel components.Microalloying also offers improved weldability, which is a critical factor in steel fabrication. The addition of elements like niobium helps refine the grain structure of steel, reducing the risk of cracking during the welding process. This not only increases the reliability of the welds but also allows for the use of higher heat input, resulting in improved productivity and cost-effectiveness.In addition to strength and weldability, microalloying improves the formability of steel. The presence of microalloying elements modifies the crystal structure of the steel, making it more resistant to deformation and improving its ability to be shaped into complex forms. This is particularly beneficial in the manufacturing of automotive components, where intricate shapes and lightweight design are highly desired.Furthermore, microalloying enhances the toughness of steel, making it more resistant to brittle fracture. The addition of microalloying elements helps to refine the microstructure of the steel, reducing the size of the grains and improving the distributionof precipitates. This leads to increased resistance to crack propagation and improved toughness, making microalloyed steel suitable for applications in harsh environments.Apart from the mechanical properties, microalloying also offers environmental benefits. The use of microalloyed steel reduces the need for energy-intensive processes, such as heat treatment, to achieve desired mechanical properties. This not only saves energy but also reduces greenhouse gas emissions, making microalloyed steel a more sustainable choice compared to traditional alloying techniques.In conclusion, microalloying has a significant impact on steel, enhancing its strength, weldability, formability, and toughness. The addition of microalloying elements, such as niobium, vanadium, or titanium, in small quantities improves the mechanical properties of steel without sacrificing its ductility. Furthermore, microalloyed steel offers environmental benefits, making it a sustainable choice for various applications. The use of microalloying has revolutionized the steel industry, enabling the production of high-performance steel with improved properties and reduced production costs.。
The effect of pressure on materials properties

The effect of pressure on materialsproperties材料是人类生活中不可或缺的一部分,它们具有多种功能,如支撑和保护结构、储存和分离物质、传递和转换能量等。
材料的特性与压力密切相关,这是因为压力可引起材料内部结构与组成的变化,从而影响其力学、物理、化学和生物性质。
一、力学性质在力学方面,压力对材料的影响主要表现为两个方面:一是压力下材料的承载力,即抗拉抗压性;二是压力下的形变能力,即材料的韧性和脆性。
抗拉抗压性一般指材料在单向应力(拉伸或压缩)下的应变状况。
随着压力的不断增加,材料内部分子间距将变得更小,其内在分子力相互作用也将随之增加。
在一定压力范围内,材料的抗拉抗压性能随之增强,而超出这一范围则会引起塑性变形和裂纹扩展,从而失去承载能力。
韧性是描述材料在受应力时能够承受塑性变形的能力。
材料韧性越高,意味着其在受外力的冲击后能够保持变形,而不破裂或塑性变形不明显,同时能够回复原本状态。
而脆性则是指材料的抗冲击性能,即材料在外力冲击下破裂或断裂的趋势。
例如,玻璃是一种具有高硬度和脆性的材料,在受到冲击后容易破碎。
二、物理性质在物理学方面,压力会直接影响物体的体积、密度、热传导率和电导率等特性。
当物体受到压力时,其分子之间的距离将减小,导致物体的密度变化。
例如,当把一块海绵压缩成小块时,其密度将随之增加。
因此,体积与密度两个参数可以相互转换。
此外,压力还会影响材料的热传导率和电导率。
特定压力下材料的热传导性质与材料分子的振动速率有关。
随着压力的增加,分子振动速率会减缓,这将影响其热传导率。
同理,在压力下,金属的导电性能也会受到影响。
当压力过大时,会导致金属电子的难以流动,进而降低其电导率。
三、化学性质压力对化学性质的影响主要体现在材料的反应速率和反应的数量上。
当压力增加时,化学反应速率往往也随之增加。
例如,在工业生产中,许多高压反应器用于加速化学反应。
同时,在一些体积的金刚石附近,由于高压和高温的作用,常发现一些新的化学物质。
合金粘度的影响英文文献
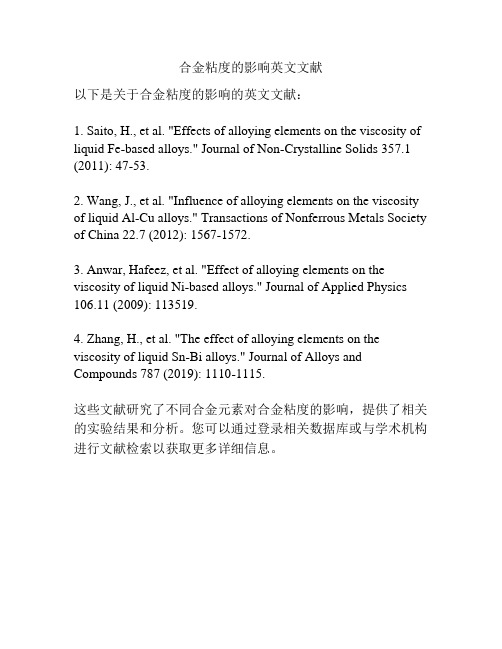
合金粘度的影响英文文献
以下是关于合金粘度的影响的英文文献:
1. Saito, H., et al. "Effects of alloying elements on the viscosity of liquid Fe-based alloys." Journal of Non-Crystalline Solids 357.1 (2011): 47-53.
2. Wang, J., et al. "Influence of alloying elements on the viscosity of liquid Al-Cu alloys." Transactions of Nonferrous Metals Society of China 22.7 (2012): 1567-1572.
3. Anwar, Hafeez, et al. "Effect of alloying elements on the viscosity of liquid Ni-based alloys." Journal of Applied Physics 106.11 (2009): 113519.
4. Zhang, H., et al. "The effect of alloying elements on the viscosity of liquid Sn-Bi alloys." Journal of Alloys and Compounds 787 (2019): 1110-111
5.
这些文献研究了不同合金元素对合金粘度的影响,提供了相关的实验结果和分析。
您可以通过登录相关数据库或与学术机构进行文献检索以获取更多详细信息。
叠加效应 英语

叠加效应 英语《The Power of the Superposition Effect》In the world of science and mathematics, the concept of the superposition effect holds great significance. It refers to the phenomenon where the combined effect of multiple factors is greater than the sum of their individual effects. This principle can be observed in various fields, from physics to economics, and has profound implications for our understanding of complex systems. In this article, we will explore the superposition effect in detail, its applications, and the importance of considering it in our daily lives.To understand the superposition effect, let’s consider a simple example. Imagine a group of people pushing a heavy object. Each person exerts a certain amount of force, and when these forces are combined, the object moves with a greater force than any individual could achieve alone. This is the essence of the superposition effect – the collective action of multiple elements results in a more significant outcome.In the field of physics, the superposition principle is widely used to describe the behavior of waves. When two or more waves interact, their amplitudes add up, creating a resultant wave with a unique pattern. This principle is crucial in understanding phenomena such as interference and diffraction, which have important applications in areas like optics and telecommunications.The superposition effect also plays a crucial role in economics. For instance, in a market, the combined actions of consumers and producers determine the overall supply and demand. When there is an increase in both consumer demand and producer supply, the market experiences a greater impact than if only one of these factors were to change. This understanding is essential for policymakers and businesses to make informed decisions and predict market trends.In the realm of human behavior, the superposition effect can be observed in various situations. For example, in a team environment, the combined skills and efforts of team members can lead to more significant achievements than if each member were to work independently. Similarly, in a social setting, the cumulative effect of individual actions can have a profound impact on the community as a whole.One of the key implications of the superposition effect is that it highlights the importance of considering multiple factors when analyzing a situation. By looking beyond individual elements and considering their interactions, we can gain a more comprehensive understanding of complex systems. This approach is particularly relevant in fields such as climate science, where the combined effects of various factors, such as greenhouse gas emissions and natural phenomena, determine the state of the climate.Furthermore, the superposition effect reminds us of the power of collective action. When individuals come together and work towards a common goal, their combined efforts can have a far-reaching impact. This is evident in social movements, where the collective voice of many can bring about significant change.The superposition effect is a powerful concept that has wide-ranging applications in various fields. It emphasizes the importance of considering the combined effects of multiple factors and the potential for collective action to create more significant outcomes. By understanding and applying this principle, we can better analyze complex systems, make informed decisions, and work towards achieving greater goals. So, the next time you encounter a situation where multiple factors are at play, remember the power of the superposition effect and its potential to shape our world.。
characteristic element

characteristic elementWhat is a characteristic element? It can be described as an element that exhibits certain unique properties or characteristics. These characteristics can vary from element to element, but generally they are what makes each element distinct from others. In science, we are often interested in exploring the characteristics of elements to better understand their nature and properties.In physics, for instance, the element hydrogen has a unique characteristic called the proton. Protons are the fundamental particles that make up atoms, and they have a positive charge. This characteristic distinguishes hydrogen from other elements, such as oxygen or nitrogen, which have different particles. Understanding the properties of protons and other particles is crucial for explaining the behavior of atoms and how they combine to form matter.Another example is the element carbon, which has numerous characteristic properties. Carbon has a unique combination of electrons and nuclei that allows it to form strong chemical bonds with other elements. This characteristic allows carbon to form the backbone of many organic compounds, including polymers and biopolymers. Understanding carbon's propertiesis essential for developing materials such as plastics, polymers, and carbon nanotubes.The characteristics of an element can have profound implications for our lives. For instance, elements such as oxygen and nitrogen are essential for life to exist, as they are necessary for the production of cellular respiration. Other elements, such as iron, play crucial roles in ourbodies as well. Understanding the characteristics of these elements and their interactions with other substances is essential for developing effective medical treatments and nutritional supplements.The study of characteristic elements is also crucial for the development of technology. For instance, certain elements, such as silicon and germanium, are critical for the manufacture of semiconductors and transistors, which are essential for modern electronic devices. Understanding the characteristics of these elements and their interactions with other materials is fundamental to the development of next-generation electronics and other advanced technologies.Moreover, characteristic elements are also important for environmental studies and sustainability. For instance,certain elements such as phosphorus and nitrogen can behighly mobile in soil and water systems, leading to environmental pollution and ecological damage. Understanding these elements' characteristics and their interactions with other substances is crucial for developing effectiveenvironmental management strategies and mitigating environmental damage.In conclusion, characteristic elements play a crucial role in our understanding of nature and the world around us. They have unique properties that make them distinct from other elements, and these properties can have profound implications for our lives, both in terms of our physical well-being and in terms of technological advancements and environmental sustainability. Therefore, studying characteristic elements is essential for gaining a deeper understanding of the world and for developing effective solutions to address our challenges.In the future, it may be possible to use advanced techniques such as quantum computing to further explore the characteristics of elements and unlock their potential for new applications and technologies. Additionally, advances in materials science and nanotechnology may lead to the development of new materials that are optimized for specific applications based on the characteristics of their constituent elements. Understanding characteristic elements will continue to be an essential part of scientific exploration and innovation in the decades to come.。
Effect of Alloying Elements on the Mechanical Properties of the Stable Austenitic Stainless Steel
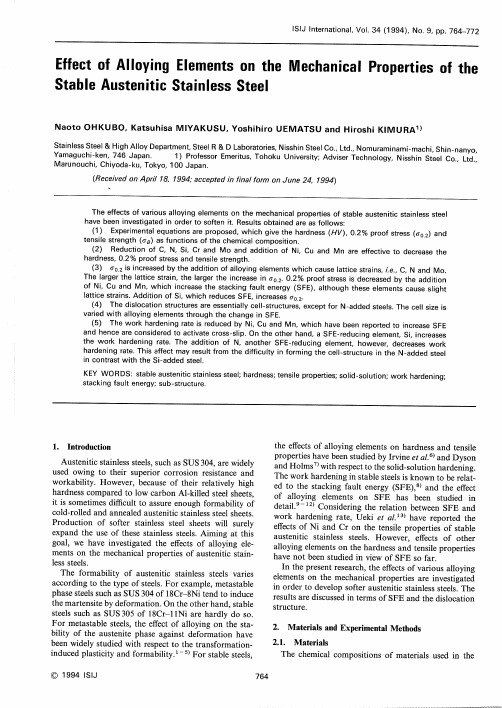
-----
1050'CXlmin
0.004 o,004Biblioteka 0,003 o,ool0.09
0,026 0.001 12.53 16.88 0.022 0.001
The effects
of various alloying
in
have been investigated
)
order to soften
N
(5) The work hardening rate is reduced by Ni, Cu and Mn, which have been reported to increase SFE and hence are considered to activate cross-slip. Onthe other hand, a SFE-reducing element, Si, increases the work hardening rate. The addition of N, another SFE-reducing element, however, decreases work hardening rate. This **ffect mayresult from the ditficulty in forming the cell-structure in the N-added steel
Table
SampleNo.
1.
Chemical compositions of investigated
Si
o.09
materials.
C
0.003
Mn P
0.80
o.77
s
Ni
Cr
Mo Cu N
effects of alloying elements
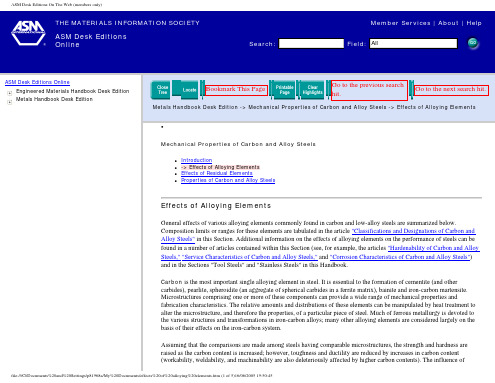
but to a lesser degree than does carbon; the amount of increase depends on the carbon content. Manganese has a strong effect on increasing the hardenability of a steel. Manganese has less of a tendency toward macrosegregation than any of the common elements. Steels with more than 0.60% Mn cannot be readily rimmed. Manganese is beneficial to surface quality in all carbon ranges (with the exception of extremely low-carbon rimmed steels.
Mechanical Properties of Carbon and Alloy Steels
q q q q
Introduction -> Effects of Alloying Elements Effects of Residual Elements Properties of Carbon and Alloy Steels
Manganese is normally present in all commercial steels. It is important in the manufacture of steel because it deoxidizes the
melt and facilitates hot working of the steel by reducing the susceptibility to hot shortness. Manganese also combines with sulfur to form manganese sulfide stringers, which improve the machinability of steel. It contributes to strength and hardness,
元素替代对LaO0.5F0.5Bi(Se1
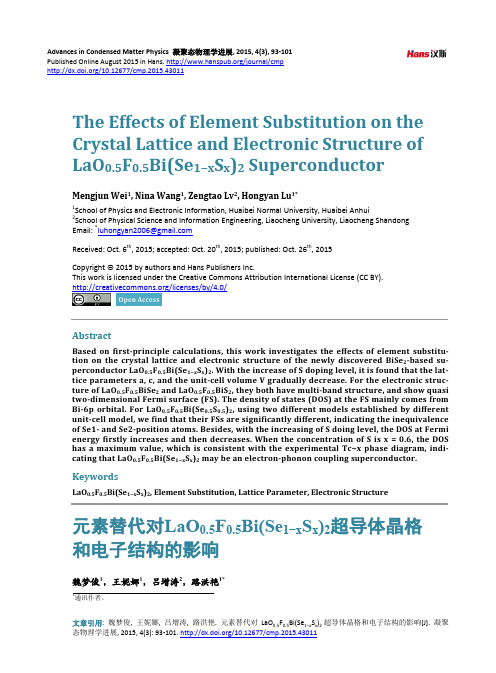
Advances in Condensed Matter Physics 凝聚态物理学进展, 2015, 4(3), 93-101Published Online August 2015 in Hans. /journal/cmp/10.12677/cmp.2015.43011The Effects of Element Substitution on theCrystal Lattice and Electronic Structure ofLaO0.5F0.5Bi(Se1−x S x)2 SuperconductorMengjun Wei1, Nina Wang1, Zengtao Lv2, Hongyan Lu1*1School of Physics and Electronic Information, Huaibei Normal University, Huaibei Anhui2School of Physical Science and Information Engineering, Liaocheng University, Liaocheng ShandongEmail: *luhongyan2006@Received: Oct. 6th, 2015; accepted: Oct. 20th, 2015; published: Oct. 26th, 2015Copyright © 2015 by authors and Hans Publishers Inc.This work is licensed under the Creative Commons Attribution International License (CC BY)./licenses/by/4.0/AbstractBased on first-principle calculations, this work investigates the effects of element substitu-tion on the crystal lattice and electronic structure of the newly discovered BiSe2-based su-perconductor LaO0.5F0.5Bi(Se1−x S x)2. With the increase of S doping level, it is found that the lat- tice parameters a, c, and the unit-cell volume V gradually decrease. For the electronic struc-ture of LaO0.5F0.5BiSe2 and LaO0.5F0.5BiS2, they both have multi-band structure, and show quasi two-dimensional Fermi surface (FS). The density of states (DOS) at the FS mainly comes from Bi-6p orbital. For LaO0.5F0.5Bi(Se0.5S0.5)2, using two different models established by different unit-cell model, we find that their FSs are significantly different, indicating the inequivalence of Se1- and Se2-position atoms. Besides, with the increasing of S doing level, the DOS at Fermi energy firstly increases and then decreases. When the concentration of S is x = 0.6, the DOS has a maximum value, which is consistent with the experimental Tc~x phase diagram, indi-cating that LaO0.5F0.5Bi(Se1−x S x)2 may be an electron-phonon coupling superconductor.KeywordsLaO0.5F0.5Bi(Se1−x S x)2, Element Substitution, Lattice Parameter, Electronic Structure元素替代对LaO0.5F0.5Bi(Se1−x S x)2超导体晶格和电子结构的影响魏梦俊1,王妮娜1,吕增涛2,路洪艳1**通讯作者。
Effect of yttrium on the glass-forming ability of Fe–Cr–Mo–C–B
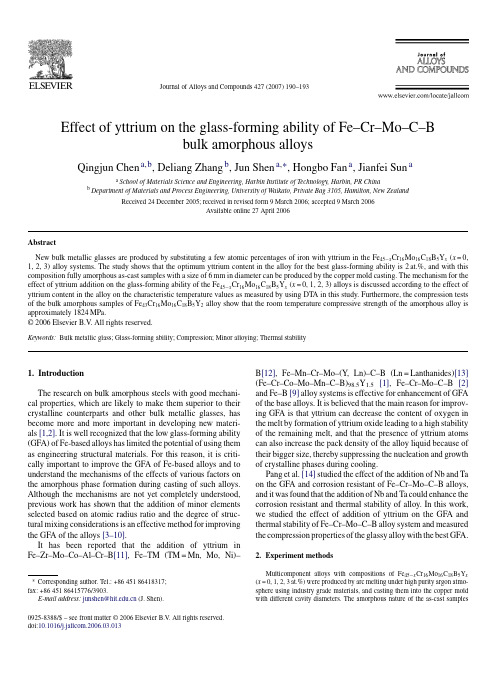
Journal of Alloys and Compounds427(2007)190–193Effect of yttrium on the glass-forming ability of Fe–Cr–Mo–C–Bbulk amorphous alloysQingjun Chen a,b,Deliang Zhang b,Jun Shen a,∗,Hongbo Fan a,Jianfei Sun aa School of Materials Science and Engineering,Harbin Institute of Technology,Harbin,PR Chinab Department of Materials and Process Engineering,University of Waikato,Private Bag3105,Hamilton,New ZealandReceived24December2005;received in revised form9March2006;accepted9March2006Available online27April2006AbstractNew bulk metallic glasses are produced by substituting a few atomic percentages of iron with yttrium in the Fe45−x Cr16Mo16C18B5Y x(x=0, 1,2,3)alloy systems.The study shows that the optimum yttrium content in the alloy for the best glass-forming ability is2at.%,and with this composition fully amorphous as-cast samples with a size of6mm in diameter can be produced by the copper mold casting.The mechanism for the effect of yttrium addition on the glass-forming ability of the Fe45−x Cr16Mo16C18B5Y x(x=0,1,2,3)alloys is discussed according to the effect of yttrium content in the alloy on the characteristic temperature values as measured by using DTA in this study.Furthermore,the compression tests of the bulk amorphous samples of Fe43Cr16Mo16C18B5Y2alloy show that the room temperature compressive strength of the amorphous alloy is approximately1824MPa.©2006Elsevier B.V.All rights reserved.Keywords:Bulk metallic glass;Glass-forming ability;Compression;Minor alloying;Thermal stability1.IntroductionThe research on bulk amorphous steels with good mechani-cal properties,which are likely to make them superior to their crystalline counterparts and other bulk metallic glasses,has become more and more important in developing new materi-als[1,2].It is well recognized that the low glass-forming ability (GFA)of Fe-based alloys has limited the potential of using them as engineering structural materials.For this reason,it is criti-cally important to improve the GFA of Fe-based alloys and to understand the mechanisms of the effects of various factors on the amorphous phase formation during casting of such alloys. Although the mechanisms are not yet completely understood, previous work has shown that the addition of minor elements selected based on atomic radius ratio and the degree of struc-tural mixing considerations is an effective method for improving the GFA of the alloys[3–10].It has been reported that the addition of yttrium in Fe–Zr–Mo–Co–Al–Cr–B[11],Fe–TM(TM=Mn,Mo,Ni)–∗Corresponding author.Tel.:+8645186418317;fax:+8645186415776/3903.E-mail address:junshen@(J.Shen).B[12],Fe–Mn–Cr–Mo–(Y,Ln)–C–B(Ln=Lanthanides)[13] (Fe–Cr–Co–Mo–Mn–C–B)98.5Y1.5[1],Fe–Cr–Mo–C–B[2] and Fe–B[9]alloy systems is effective for enhancement of GFA of the base alloys.It is believed that the main reason for improv-ing GFA is that yttrium can decrease the content of oxygen in the melt by formation of yttrium oxide leading to a high stability of the remaining melt,and that the presence of yttrium atoms can also increase the pack density of the alloy liquid because of their bigger size,thereby suppressing the nucleation and growth of crystalline phases during cooling.Pang et al.[14]studied the effect of the addition of Nb and Ta on the GFA and corrosion resistant of Fe–Cr–Mo–C–B alloys, and it was found that the addition of Nb and Ta could enhance the corrosion resistant and thermal stability of alloy.In this work, we studied the effect of addition of yttrium on the GFA and thermal stability of Fe–Cr–Mo–C–B alloy system and measured the compression properties of the glassy alloy with the best GFA.2.Experiment methodsMulticomponent alloys with compositions of Fe45−x Cr16Mo16C18B5Y x (x=0,1,2,3at.%)were produced by arc melting under high purity argon atmo-sphere using industry grade materials,and casting them into the copper mold with different cavity diameters.The amorphous nature of the as-cast samples0925-8388/$–see front matter©2006Elsevier B.V.All rights reserved. doi:10.1016/j.jallcom.2006.03.013Q.Chen et al./Journal of Alloys and Compounds 427(2007)190–193191was checked using X-ray diffraction (XRD)(Philips Xpert system with Cu K ␣radiation)and transmission electron microscopy (TEM)(Philips CM200).The specimens for TEM observation were prepared by mechanical polishing samples to 100m in thickness followed by further thinning in a chemical solution.The as-cast amorphous samples were also analysed using difference thermal analysis (DTA)(Perkin-Elmer thermal analysis system)at a heating rate of 20K/min.The uniaxial compression tests of as-cast samples were performed using cylindrical specimen with a diameter of 5mm and a length of 10mm.The ends of as-cast samples were carefully polished to make them flat and parallel.The initial strain rate for the compressive tests was 1×10−3s −1.3.Results and discussionFig.1shows the XRD patterns of the as-cast Fe 45−x Cr 16Mo 16C 18B 5Y x (x =1,2,3at.%)alloy samples with diameters of 3or 6mm under the facility conditions of this study.It was impossible to fabricate bulk amorphous sample out of the base Fe 45Cr 16Mo 16C 18B 5alloy without yttrium addition,even with a sample diameter of 1mm.With the substitution of 1at.%iron by yttrium,a bulk amorphous rod of 2mm in diameter can be produced using the copper mold casting technique.However,for this alloy,the XRD pattern (Fig.1)of the as-cast rod of 3mm in diameter shows some sharp peaks corresponding to the -Fe 2C crystalline phase on the main halo peak,which indi-cates that the structure of 3mm rod consists of a substantial amount of -Fe 2C crystalline phase in addition to the amor-phous phase.When 2at.%of iron is substituted by yttrium,the XRD pattern (Fig.1)of as-cast rod as large as 6mm in diame-ter only shows a halo peak without sharp peaks corresponding to crystalline phases.In the meantime,when 3at.%of iron is substituted by yttrium,the XRD pattern (Fig.1)of a rod of 3mm in diameter exhibits some sharp peaks corresponding to -Fe 2C,YB 6and other unknown crystalline phases.Based on above results,it can be concluded that the addition of yttrium of up to 2at.%in place of iron can lead to a significantly improved GFA in the Fe 45−x Cr 16Mo 16C 18B 5Y x alloy system.To fur-ther confirm the amorphous structure of Fe 43Cr 16Mo 16C 18B 5Y 2samples,TEM examination was performed.As shown in Fig.2,Fig.1.XRD patterns of as-cast Fe 45−x Cr 16Mo 16C 18B 5Y x (x =1,2,3at.%)alloysamples.Fig.2.TEM bright-field image and the corresponding selected area electron diffraction pattern (inset)of the Fe 43Cr 16Mo 16C 18B 5Y 2amorphous alloy sam-ple with a diameter of 6mm.the bright-field TEM image shows that there is no contrast of crystalline phases,and the corresponding selected area electron diffraction pattern only contains a single wide ring,which is a typical characteristic of amorphous structure.So the structure of Fe 43Cr 16Mo 16C 18B 5Y 2rod with 6mm in diameter is fully amorphous according to the experiments results of XRD and TEM.These results prove that the addition of yttrium can effec-tively suppress the crystalline phase formation to enhance the GFA of the Fe–Cr–Mo–C–B alloy system.Similar results have also been obtained in other Fe-based bulk glassy alloy systems [9,11,12].It is worthwhile to note that the optimum content of yttrium in Fe-based alloys having the best GFA usually lies between 2and 5at.%and the yttrium content beyond this range results in a decrease of GFA.The reason seems to be associated with the chemical concentration fluctuation required by for-mation of crystalline phases during cooling of the alloy liq-uid.The yttrium content of the alloy liquid in this range may make it difficult to satisfy the equilibrium composition require-ment for possible formation of crystalline phases.In the mean-while,it is the alloy liquid with a composition in this range can have a high atomic packing density due to the differ-ences of radii of Y (2.27˚A),Mo (2.01˚A),Cr (1.85˚A),Fe (1.72˚A),B (1.17˚A)andC (0.91˚A)atoms,which can block the long-range atomic diffusion,thereby increase the difficulty of nucleation of crystalline phases and facilitate the formation of amorphous phase.The further more detailed investigation into the mechanisms of the effects of yttrium addition on the amorphous phase formation should be carried out at atomic192Q.Chen et al./Journal of Alloys and Compounds427(2007)190–193Fig.3.DTA traces of the Fe45−x Cr16Mo16C18B5Y x(x=1,2,3at.%)alloys at a heating rate of20K/min.scale by high resolution transmission electron microscopy (HRTEM).Fig.3shows the DTA traces of the as-cast samples of the Fe45−x Cr16Mo16C18B5Y x(x=1,2,3at.%)alloys.The values of characteristic temperatures including glass transition tem-perature(T g),crystallization onset temperature(T x),melting temperature(T m)and liquidus temperature(T l)(as shown by the arrows in Fig.3)are listed in Table1.From Fig.3,it can be seen that there is not much difference in the lower temperature sections of the three DTA traces corresponding to the different contents of yttrium,but the numbers and shapes of melting peaks in the higher temperature sections of the three DTA traces vary significantly.The DTA trace of the alloy with1at.%yttrium and that of the alloy with3at.%yttrium both exhibit two melt-ing peaks,but the DTA trace of the alloy with2at.%yttrium has only one melting peak.This implies that the composition of the alloy with2at.%yttrium is close to an eutectic point of the multicomponent system leading to a higher GFA,compared to other paring the characteristic temperature values as listed in Table1,it can be observed that the liquidus temperatures (T l)of the alloy with2at.%yttrium is the lowest among four Fe45−x Cr16Mo16C18B5Y x(x=0,1,2,3at.%)alloys.This means that the melt of the alloy with2at.%yttrium prior to solidifi-cation has the highest stability against crystallization,enabling the highest GFA.Moreover,the values of several GFA param-eters,namely,T rg(T g/T l)[15],γ(T x/(T l+T g))[16,17]andδ(T x/(T l−T g))[18]were calculated using the chacteristic tem-Table1Values of the characteristic temperatures and the GFA parameters of the Fe45−x Cr16Mo16C18B5Y x(x=0,1,2,3at.%)alloysAddition of YelementT g(K)T x(K)T m(K)T l(K)T rg(K)γδ0––14061637–––1868957139316210.5350.384 1.271 2864950140815560.5550.393 1.373 3872950138916200.5380.381pressive stress–strain curve of the as-cast Fe43Cr16Mo16C18B5Y2 amorphous alloy sample of5mm in diameter.perature data and also listed in Table1.It is evident that thealloy with2at.%yttrium has the highest values of T rg,γ,and δ,confirming that this alloy indeed has the highest GFA among the Fe45−x Cr16Mo16C18B5Y x(x=0,1,2,3at.%)alloys.To examine the mechanical behavior of the new bulk glassyFe43Cr16Mo16C18B5Y2alloy,uniaxial compression tests wereperformed at room temperature.Fig.4shows the compressionstress–stain curve of Fe43Cr16Mo16C18B5Y2amorphous rodwith a diameter of5mm.It can be observed that there is noplastic deformation prior to thefinal fracture at an elastic strainof1.7%,which implies that this glassy alloy is brittle in nature.The compressive fracture strength of the alloy was measured tobe1824MPa which is much lower than those of other Fe-basebulk metallic glasses[19–21].This might be due to the differentbond nature among the atoms of the constitute elements[22].Serrateflow was observed during deformation of the bulk glassyalloy as shown by the plateaus of the compressive stress–straincurve(circles shown in Fig.4).This serrateflow might resultfrom the propagation of shear bands during compression.Thefracture surfaces of the samples show that the mode of compres-sion fracture is purely brittle on macroscopic scale,but featuresindicating a certain amount of microscopic plastic deformationare obviously visible.The detailed investigation on the fracturebehaviors of the alloys of this study will be presented elsewhere.4.ConclusionsIt has been clearly demonstrated that proper substitution ofa small fraction of iron by yttrium in the Fe45Cr16Mo16C18B5alloy system can significantly enhance the glass-forming ability.The optimum composition in terms of the highest GFA in the Fe-based alloy system of this study is the alloy with an yttrium con-tent of2at.%.Fully amorphous rod with a diameter of up to6mmcan be produced by the copper mold casting for the alloy withthe optimum composition.The compressive fracture strengthand elastic strain to fracture of the amorphous alloy with2at.%yttrium are approximately1824MPa and1.7%,respectively.Q.Chen et al./Journal of Alloys and Compounds427(2007)190–193193AcknowledgementOne of the authors(J.Shen)would like to acknowledge the financial support by the Program for New Century Excellent Talents in University(China).References[1]Z.P.Lu,C.T.Liu,J.R.Thompson,W.D.Porter,Phys.Rev.Lett.92(2004)245503–245511.[2]V.Ponnambalam,S.J.Poon,G.J.Shiflet,J.Mater.Res.19(2004)1320.[3]L.Ma,L.Wang,T.Zhang,A.Inoue,Mater.Res.Bull.34(1999)915.[4]J.K.Lee,W.T.Kim,D.H.Kim,Mater.Lett.57(2003)1514.[5]J.K.Lee,D.H.Bae,S.Yi,W.T.Kim,D.H.Kim,J.Non-Cryst.Solids333(2004)212.[6]L.Q.Xing,P.Ochin,J.Bigot,J.Non-Cryst.Solids205–207(1996)637.[7]E.S.Park,H.G.Kang,W.T.Kim,D.H.Kim,J.Non-Cryst.Solids279(2001)154.[8]G.Y.Yuan,A.Inoue,J.Alloys Compd.387(2005)134.[9]J.Zhang,H.Tan,Y.P.Feng,Y.Li,Scripta Mater.53(2005)183.[10]Z.P.Lu,C.T.Liu,J.Mater.Sci.39(2004)3965.[11]Z.P.Lu,C.T.Liu,W.D.Porter,Appl.Phys.Lett.83(2003)2581.[12]J.H.Kim,J.S.Park,E.Fleury,W.T.Kim,D.H.Kim,Mater.Trans.45(2004)2770.[13]V.Ponnambalam,S.J.Poon,G.J.Shiflet,J.Mater.Res.19(2004)3046.[14]S.J.Pang,T.Zhang,K.Asami,A.Inoue,Mater.Trans.42(2001)376.[15]D.Turnbull,Contemp.Phys.10(1969)473.[16]Z.P.Lu,C.T.Liu,Acta Mater.50(2002)3501.[17]Z.P.Lu,C.T.Liu,Phys.Rev.Lett.91(2003)115505–115511.[18]Q.J.Chen,J.Shen,H.B.Fan,J.F.Sun,Y.J.Huang,D.G.McCartney,Chin.Phys.Lett.22(2005)1736.[19]M.Stoica,J.Eckert,S.Roth,Z.F.Zhang,L.Schultz,W.H.Wang,Inter-metallics13(2005)764.[20]J.Shen,Q.J.Chen,J.F.Sun,H.B.Fan,G.Wang,Appl.Phys.Lett.86(2005)151907–151911.[21]Q.J.Chen,H.B.Fan,L.Ye,S.Ringer,J.F.Sun,J.Shen,D.G.McCartney,Mater.Sci.Eng.A402(2005)188.[22]F.R.De Boer,R.Boom,W.C.M.Mattens,A.R.Miedema,A.K.Niessen,Cohesions in Metals,Amsterdam,The Netherlands,North-Holland, 1988.。
钢材里加入合金元素的作用
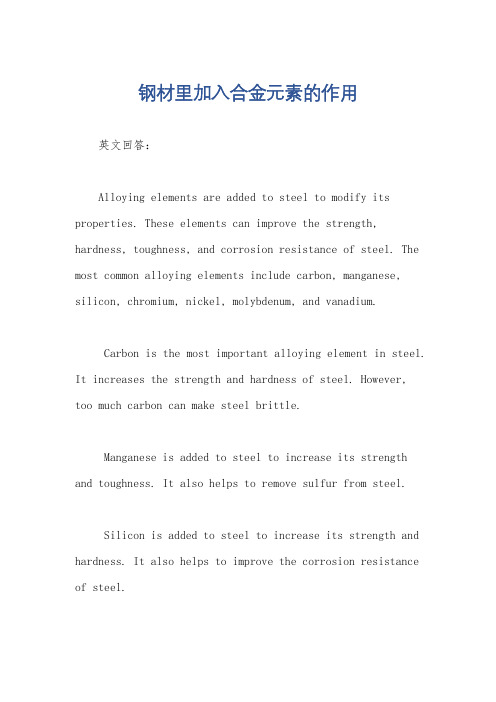
钢材里加入合金元素的作用英文回答:Alloying elements are added to steel to modify its properties. These elements can improve the strength, hardness, toughness, and corrosion resistance of steel. The most common alloying elements include carbon, manganese, silicon, chromium, nickel, molybdenum, and vanadium.Carbon is the most important alloying element in steel. It increases the strength and hardness of steel. However, too much carbon can make steel brittle.Manganese is added to steel to increase its strength and toughness. It also helps to remove sulfur from steel.Silicon is added to steel to increase its strength and hardness. It also helps to improve the corrosion resistance of steel.Chromium is added to steel to increase its strength and corrosion resistance. It is also used to make stainless steel.Nickel is added to steel to increase its strength and toughness. It is also used to make stainless steel.Molybdenum is added to steel to increase its strength and toughness. It is also used to improve the high-temperature strength of steel.Vanadium is added to steel to increase its strength and toughness. It is also used to improve the fatigue strength of steel.The amount of alloying elements added to steel depends on the desired properties of the steel. For example, steel that is used for structural applications requires a high strength and toughness. Steel that is used for corrosion-resistant applications requires a high chromium content.中文回答:合金元素添加到钢中是为了改变其性能。
The Properties and Uses of Ferromagnetic Materials
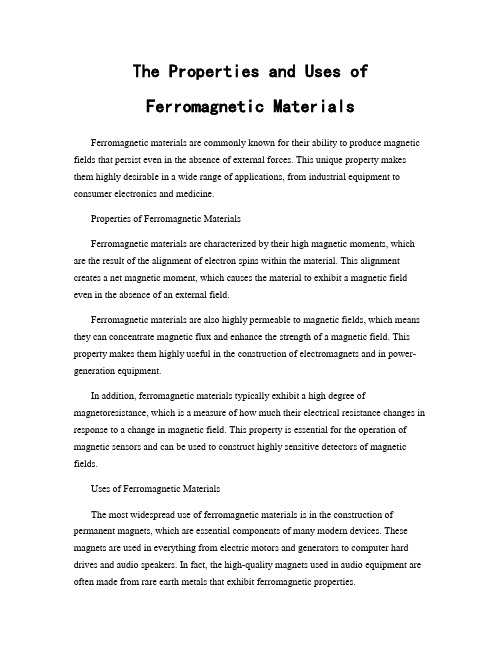
The Properties and Uses ofFerromagnetic MaterialsFerromagnetic materials are commonly known for their ability to produce magnetic fields that persist even in the absence of external forces. This unique property makes them highly desirable in a wide range of applications, from industrial equipment to consumer electronics and medicine.Properties of Ferromagnetic MaterialsFerromagnetic materials are characterized by their high magnetic moments, which are the result of the alignment of electron spins within the material. This alignment creates a net magnetic moment, which causes the material to exhibit a magnetic field even in the absence of an external field.Ferromagnetic materials are also highly permeable to magnetic fields, which means they can concentrate magnetic flux and enhance the strength of a magnetic field. This property makes them highly useful in the construction of electromagnets and in power-generation equipment.In addition, ferromagnetic materials typically exhibit a high degree of magnetoresistance, which is a measure of how much their electrical resistance changes in response to a change in magnetic field. This property is essential for the operation of magnetic sensors and can be used to construct highly sensitive detectors of magnetic fields.Uses of Ferromagnetic MaterialsThe most widespread use of ferromagnetic materials is in the construction of permanent magnets, which are essential components of many modern devices. These magnets are used in everything from electric motors and generators to computer hard drives and audio speakers. In fact, the high-quality magnets used in audio equipment are often made from rare earth metals that exhibit ferromagnetic properties.Ferromagnetic materials are also frequently used in the construction of electromagnets, which are used in a wide range of industrial and scientific applications. Electromagnets are often employed in electric motors and generators, particle accelerators, and magnetic resonance imaging (MRI) machines.One of the most exciting potential uses of ferromagnetic materials is in the field of spintronics, which is the study of the properties of electrons and their interactions with magnetic fields. In spintronics, ferromagnetic materials are used to manipulate the spin of electrons to create new types of electronic devices that are faster and more efficient than their conventional counterparts.Finally, ferromagnetic materials can also be used in the construction of magnetic sensors, which are essential for many scientific and industrial applications. Magnetic sensors can be used to measure magnetic fields, detect magnetic anomalies in materials, and even to create images of magnetic fields in biological tissues.ConclusionFerromagnetic materials are among the most versatile and useful substances in modern technology. With their unique properties, they play a critical role in a wide range of applications, from consumer electronics to medical imaging and scientific research. As we continue to develop new materials and technologies, it is likely that we will discover even more exciting uses for these remarkable substances in the years to come.。
BS EN 10305-1-2002(上海凯蔚机电科技) (1)

Effect of Chemical Elements in Steel 钢铁中化学元素的作用Have you ever wondered what all those elements listed on a materials test report really mean? Read on!你有想知道在材料检测报告中列出来的元素真正是什么意思吗?请读下去。
Carbon - is generally considered to be the most important alloying element in steel and can be present up to 2% (although most welded steels have less than 0.5%). Increased amounts of carbon increase hardness and tensile strength, as well as response to heat treatment (hardenability). Increased amounts of carbon will reduce weldability.碳-----通常被认为是在钢中最重要的合金元素的碳- 最高含量可达2%(虽然大多数的焊接钢碳的含量小于0.5%)。
增加碳的含量能增加钢铁的硬度和拉伸强度,以及响应于热处理(淬透性)。
增加的碳量会降低可焊性。
Sulfur - is usually an undesirable impurity in steel rather than an alloying element. In amounts exceeding 0.05% it tends to cause brittleness and reduce weldability. Alloying additions of sulfur in amounts from 0.10% to 0.30% will tend to improve the machinability of a steel. Such types may be referred to as "resulfurized" or "free-machining". Free-machining alloys are not intended for use where welding is required.硫- 与其说是一种合金元素,倒不如说是通常钢铁中的不希望有的杂质。
Alloying Effects
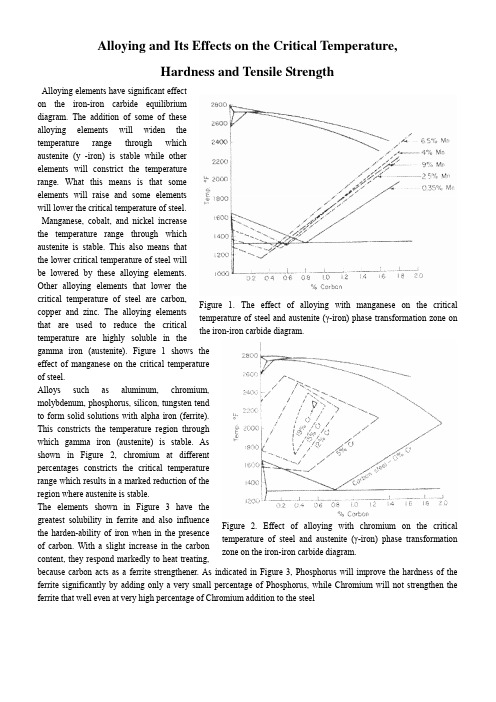
Alloying and Its Effects on the Critical Temperature,Hardness and Tensile StrengthAlloying elements have significant effecton the iron-iron carbide equilibriumdiagram. The addition of some of these alloying elements will widen the temperature range through whichaustenite (y -iron) is stable while otherelements will constrict the temperaturerange. What this means is that someelements will raise and some elementswill lower the critical temperature of steel.Manganese, cobalt, and nickel increasethe temperature range through whichaustenite is stable. This also means thatthe lower critical temperature of steel willbe lowered by these alloying elements.Other alloying elements that lower thecritical temperature of steel are carbon, copper and zinc. The alloying elements that are used to reduce the critical temperature are highly soluble in thegamma iron (austenite). Figure 1 shows theeffect of manganese on the critical temperatureof steel.Figure 1. The effect of alloying with manganese on the critical temperature of steel and austenite (γ-iron) phase transformation zone on the iron-iron carbide diagram. Alloys such as aluminum, chromium,molybdenum, phosphorus, silicon, tungsten tendto form solid solutions with alpha iron (ferrite).This constricts the temperature region throughwhich gamma iron (austenite) is stable. Asshown in Figure 2, chromium at differentpercentages constricts the critical temperaturerange which results in a marked reduction of theregion where austenite is stable.The elements shown in Figure 3 have thegreatest solubility in ferrite and also influence the harden-ability of iron when in the presence of carbon. With a slight increase in the carbon content, they respond markedly to heat treating,because carbon acts as a ferrite strengthener. As indicated in Figure 3, Phosphorus will improve the hardness of the ferrite significantly by adding only a very small percentage of Phosphorus, while Chromium will not strengthen the ferrite that well even at very high percentage of Chromium addition to the steelFigure 2. Effect of alloying with chromium on the critical temperature of steel and austenite (γ-iron) phase transformation zone on the iron-iron carbide diagram.Figure 4 shows the effect offurnace cooling vs. air coolingon the tensile strength of steelfor three different percentagesof carbon in the presence ofchromium. As this figureindicates, furnace cooling hasvery little effect on the tensilestrength of the material.Figure 3. The effect of various alloying elements on the hardness of steel.Figure 4. Effect of different percentages of carbon on the tensile strength of steel in the presence of chromium. The addition of chromium doesnot change the tensile strengthproperties when the steel iscooled in the furnace. If thesame steels are air cooled at thesame rate, the slope of thecurves increases significantlywhich means that a slightincrease in the chromiumcontent increases the strengthdrastically when air-cooling isapplied.。