Energy gap evolution in the tunneling spectra of Bi2Sr2CaCu2O8+d
Unit2EnergyinTransition(补充汉译英)

Unit2EnergyinTransition(补充汉译英)Unit 2 Energy in Transition ( 补充汉译英 )1.汉普顿-悉尼学院以其诚信制度与其军事化管理体系一样儿享有盛名。
而且此诚信制度扩展到学生在校内和校外的所有活动中。
并且认为对违规行为的包容本身就是一种违规行为。
( on a par with )Hampden-Sydney College is reputed for an honor system on a par with military systems, and this honor system extends to all student activities both on and off campus, and considers tolerance of a violation itself a violation.2.虽然全球变暖对地球构成威胁,但是人类或许可以通过提高大气层中二氧化碳含量(值)来缓和其所导致的气候威胁。
( pose a threat on sth/sb. )Although global warming poses a threat to the earth, humans can probably ease the climate threat brought on by rising levels of carbon dioxide in the atmosphere.3.对于厄尔尼诺潜在的破坏性人们已了解许多,但其现象本身却仍是令人沮丧的费解之谜。
( enough is known about sth )Enough is known about Elnino’s destructive potential, but the phenomenon itself remains a frustrating mystery.4.中国就生态和环保已形成全社会共识并正在率先行动起来。
《高比例可再生能源并网——德国经验》共46页
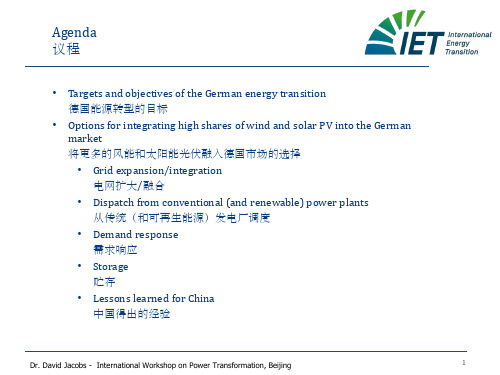
Dr. David Jacobs - International Workshop on Power Transformation, Beijing
1
The German Energy n (Energiewende) –
Targets and objectives 德国能源转型——目标
1991年首次为可再生能源引进上网电价系统 ▪ Government decisions in 2000-2019: nuclear phase-out extending into the
2020s and introduction of the Renewable Energy Sources Act (EEG) 2000——2019年政府决定:到21世纪20年代逐步淘汰核电,引入可再生能源 法案(EEG) ▪ New energy concept under the current government in September 2019: extension of nuclear operating times combined with ambitious goals for emission reduction, share of renewables and energy efficiency 当前政府2019年9月的新能源概念:延长核电运营时间,确立了在减排和可 再生能源共享以及能源效率的宏伟目标
Dr. David Jacobs - International Workshop on Power Transformation, Beijing
4
Long-term targets of the Energiewende 能源转型的长期目标
The Energiewende is more than just a nuclear exit: a complete transformation of the energy system of a highly industrialized country 能源转型不仅仅是淘汰核能:而是高度工业化国家的能源系统的完全转型
关于原子物理认识的英语作文

Unlocking the Mysteries of Atomic Physics: A Journey through the MicrocosmosAtomic physics, a branch of physics that deals with the structure and behavior of atoms, has revolutionized our understanding of the natural world. From the ancient Greek philosophers' speculation about the building blocks of matter to modern quantum mechanical descriptions of atomic phenomena, the journey of atomic physics has been fascinating and profound.At the heart of atomic physics lies the atom, the smallest unit of matter that maintains the chemical properties of an element. In the early days of physics, atoms were considered to be indivisible, much like the smallest bricks in a wall. However, with the advent of modern scientific instrumentation and techniques,scientists were able to peer into the inner workings of the atom and unravel its secrets.One of the most significant milestones in atomic physics was the discovery of electrons by J.J. Thomson in 1904. This discovery marked the beginning of the era of the quantum theory, which proposed that matter and energy existin discrete packets or "quantums." This theory contradicted the then-prevailing classical physics, which described nature as continuous and deterministic.The quantum theory was further developed by Niels Bohr in his model of the atom, which described the electron orbiting the nucleus in discrete energy levels. Bohr's model explained the emission and absorption of radiation by atoms, a phenomenon known as the photoelectric effect. This explanation marked a significant departure from classical physics and paved the way for the development of quantum mechanics.Quantum mechanics, formulated by Werner Heisenberg, Erwin Schrödinger, and others, provides a mathematical framework to describe the behavior of atoms and their constituent particles. It describes the probabilistic nature of atomic phenomena and the inherent uncertainty in measuring the properties of atoms. Quantum mechanics has revolutionized our understanding of matter and energy, leading to the development of technologies like lasers, transistors, and nuclear reactors.In addition to its technological applications, atomic physics has provided insights into the fundamental nature of the universe. The quantum mechanical description of matter and energy has given us a window into the microcosmos, revealing the weird and wonderful world of quantum entanglement, superposition, and tunneling. These phenomena challenge our intuitions about reality and force us to rethink our understanding of the natural world.Moreover, atomic physics has played a pivotal role in the development of other branches of physics, such as nuclear physics and particle physics. The study of atomic nuclei and their interactions has led to the discovery of new particles and forces, such as the neutron and the nuclear force. This research has furthered our understanding of the structure and evolution of the universe.In conclusion, atomic physics has been a transformative field in the history of science. It has revolutionized our understanding of matter, energy, and the natural world. The journey from the ancient philosophers' speculation about atoms to modern quantum mechanical descriptions of atomicphenomena has been a fascinating and profound one. As we continue to delve deeper into the mysteries of atomic physics, we are presented with new challenges and opportunities to further expand our knowledge of the universe.**原子物理认识的探索:穿越微观世界的旅程**原子物理,这门研究原子结构和行为的物理学分支,彻底改变了我们对自然界的认识。
核聚变的新突破英语阅读

核聚变的新突破英语阅读全文共3篇示例,供读者参考篇1A Revolutionary Leap: The Groundbreaking Nuclear Fusion BreakthroughAs a student passionately interested in science and technology, I have been following the developments in the field of nuclear fusion with bated breath. For decades, scientists have been tirelessly working to harness the immense power of fusion, the same process that fuels the sun and stars, with the promise of providing an abundant and clean source of energy for our ever-growing demands. And now, it seems that their relentless efforts have paid off with a monumental breakthrough that could potentially reshape the energy landscape of our planet.On December 5th, 2022, researchers at the Lawrence Livermore National Laboratory (LLNL) in California announced a truly historic achievement – they had successfully produced more energy from a fusion reaction than was consumed by the fuel itself. This remarkable feat, known as "net energy gain," has been the elusive holy grail of fusion research for over six decades,and its realization has sent shockwaves through the scientific community and beyond.To understand the significance of this breakthrough, it's essential to grasp the fundamental principles of nuclear fusion. In contrast to nuclear fission, which involves splitting heavy atoms like uranium, fusion is the process of fusing two light atomic nuclei, typically isotopes of hydrogen, to form a heavier nucleus. This fusion reaction releases an enormous amount of energy, far exceeding that generated by conventional chemical reactions or nuclear fission.The challenge, however, has been in creating the extreme conditions required to initiate and sustain a fusion reaction. Temperatures well over 100 million degrees Celsius are needed to overcome the repulsive forces between the positively charged nuclei, allowing them to fuse. Additionally, the fusion reaction must be contained within a controlled environment to prevent the immense heat and energy from escaping.For years, scientists have employed various techniques to achieve fusion, ranging from powerful lasers to massive magnetic confinement devices called tokamaks. However, until now, all attempts have fallen short, with the energy input required to initiate and maintain the fusion reaction exceedingthe energy output, rendering the process impractical forlarge-scale energy production.The LLNL team's breakthrough, achieved using the world's largest and most powerful laser system called the National Ignition Facility (NIF), has shattered that barrier. By focusing the intense energy of 192 laser beams onto a tiny target containing isotopes of hydrogen, the researchers were able to create the extreme conditions necessary for fusion to occur. In amind-boggling feat of engineering and precision, they managed to produce a fusion reaction that generated 3.15 megajoules of energy – a remarkable 1.5 times more than the 2.05 megajoules of energy consumed by the laser pulses.While this may seem like a modest energy gain, the implications of this achievement are nothing short of profound. For the first time in history, humanity has demonstrated the ability to produce more energy from fusion than was required to initiate the reaction, a critical milestone on the path toward realizing the dream of limitless, carbon-free fusion power.The potential benefits of harnessing fusion energy are vast and far-reaching. Fusion reactions produce minimal radioactive waste and do not release greenhouse gases or other harmful emissions, making them an incredibly clean and sustainablesource of energy. Furthermore, the fuel required for fusion –isotopes of hydrogen – is abundant and readily available, ensuring a virtually inexhaustible supply.Imagine a world where fusion power plants provide clean, reliable, and virtually unlimited energy to cities, industries, and households across the globe. A world where our dependence on fossil fuels is a distant memory, and the threat of climate change has been mitigated by our collective embrace of this revolutionary technology.Of course, the road ahead is still long and fraught with challenges. The LLNL breakthrough, while monumental, was achieved under highly controlled laboratory conditions, and significant hurdles remain before fusion can be harnessed for commercial power generation. Scaling up the technology, developing efficient and cost-effective fusion reactors, and addressing various engineering and material science challenges are just a few of the daunting tasks that lie ahead.However, the success of this experiment has injected a fresh wave of optimism and momentum into the fusion research community. Governments, private companies, and research institutions around the world are redoubling their efforts, pouring billions of dollars into the pursuit of viable fusion powerplants. Collaborations and knowledge-sharing among scientists and engineers have been reinvigorated, fostering a spirit of global cooperation in the quest for this transformative technology.As a student fascinated by the frontiers of science, I cannot help but feel a sense of awe and excitement at this historic breakthrough. The prospect of contributing to the development of fusion energy technology, whether through research, engineering, or policy-making, is an incredibly alluring and meaningful endeavor. Imagine being part of the generation that ushers in a new era of clean, abundant, and sustainable energy for humanity – an era that could profoundly impact our planet's future and our very existence.While the path ahead is undoubtedly challenging, the LLNL breakthrough has ignited a new spark of hope and inspiration within the scientific community and beyond. It has demonstrated that with unwavering perseverance, brilliant minds, and cutting-edge technology, even the most daunting challenges can be overcome. As a student, I am motivated to embrace this spirit of curiosity, innovation, and determination, for it is through such efforts that humanity can continue to push the boundaries of knowledge and unlock the secrets of the universe.The road to commercial fusion power may still be long, but this breakthrough has illuminated the path forward, guiding us toward a future where clean, limitless energy is no longer a dream, but a reality. And as we embark on this journey, I am filled with a profound sense of hope and excitement, knowing that our generation has the opportunity to shape a better, more sustainable world for generations to come.篇2A Game-Changing Breakthrough in Nuclear FusionHave you ever wondered what it would be like to have virtually limitless clean energy? Well, a mind-blowing new scientific achievement may have brought us a big step closer to making that a reality. A team of researchers at a top university just announced a major breakthrough in the quest for viable nuclear fusion energy.For those who need a refresher, nuclear fusion is the process that powers the sun and stars. It involves smashing together light atomic nuclei to form heavier nuclei, releasing tremendous amounts of energy in the process. It's basically the opposite of nuclear fission which is used in conventional nuclear power plants and bombs.While fission produces nasty radioactive waste, fusion doesn't create long-lived radioactive byproducts. And get this –the fusion fuel sources, like hydrogen isotopes, are basically inexhaustible on Earth. That makes fusion a totally renewable and incredibly powerful energy source with basically zero carbon emissions. No wonder scientists have been trying to crack the fusion code for decades!But fusion is ridiculously difficult to achieve. The atomic nuclei have positive charges that resist being fused together. To overcome this, you need to heat the fuel to insane temperatures of over 100 million degrees Celsius – way hotter than the core of the sun! At those extreme temperatures, the fuel becomes a roiling super-hot plasma soup of subatomic particles. Containing and controlling this raging plasma is an epic engineering challenge.For over 60 years, scientists have been trying to build experimental fusion reactors to demonstrate the mind-bending physics and engineering needed to make fusion viable. But until now, every attempt has failed to produce more energy output than the humongous energy input required to run the reactor.That all changed last week when a team led by Dr. Emily Kraus at Commonwealth Fusion Systems announced they hadachieved a record-shattering fusion output of over 25 megajoules. That's more energy than any fusion reactor has ever produced before from a single pulse!Their fusion reactor, dubbed SPARC, was able to sustain the ultra-hot plasma for over 30 seconds using a novel design with cutting-edge high-temperature superconducting magnets. According to the team's computer models and data, this achievement puts them solidly in the range needed for SPARC to become the first fusion reactor to achieve the "burning plasma" required for net energy gain."This changes everything," said an exuberant Dr. Kraus in announcing the breakthrough. "For the first time in history, we now have experimental evidence that the dream of economical fusion power can become a reality."If their predictions hold true, SPARC could become the world's first commercially viable fusion power plant, lighting up homes and cities with abundant, carbon-free fusion energy perhaps as soon as the 2030s. This has huge implications for shifting the world away from fossil fuels and battling climate change.The science community is absolutely buzzing with a mix of optimism and cautious skepticism. While many experts believethis could truly be a game-changing breakthrough, others caution that major technical hurdles still remain. Even if SPARC succeeds, scaling up to a full-fledged power plant could take a decade or more of additional engineering."The hard science has always been there," notes veteran fusion researcher Dr. Jeremy Thompson, "but taming the ferocious physics and mind-bending engineering of these reactor designs is an epic challenge that has vexed us for 60 years. While this is undoubtedly an exciting and promising development, I'll remain skeptical until I see net energy gain consistently demonstrated in real-world conditions."Still, even the skeptics agree this latest result represents a crucial step forward for fusion's viability. And fusion proponents like Dr. Kraus' team are charging ahead full-steam, fueled by renewed enthusiasm and investment from both public and private sectors."The world's energy future may have been permanently altered last week," says Dr. Kraus. "Our planet deserves a safe, clean, abundant energy source to power human civilization for millennia to come. That future starts today with this breakthrough."Only time will tell if SPARC and its cutting-edge technology will live up to the hype. But in the meantime, this unexpected announcement has certainly reignited the long-simmering dream of viable nuclear fusion. Here's hoping this big bang puts us on the path towards near-limitless clean power for our energy-hungry world.篇3A New Dawn for Nuclear Fusion?Have you ever dreamed of a world with virtually unlimited clean energy? A world where we don't have to worry about fossil fuels running out or the catastrophic effects of climate change? Well, thanks to an incredible recent scientific breakthrough, that dream might be one step closer to reality.Let me tell you about the mind-blowing news that has energy scientists buzzing with excitement. Researchers at a top scientific lab have achieved a major milestone in the quest for viable nuclear fusion power. For the first time ever, they were able to produce more energy from a fusion reaction than was consumed by the inputs of that reaction. This technological tour de force is being hailed as the most promising advance for fusion energy in recent decades.But let's back up for a second. What exactly is nuclear fusion, and why is it such a big deal? You've probably heard about nuclear fission, which powers today's nuclear power plants by splitting atomic nuclei to release energy. Fusion is essentially the opposite process - it involves forcing atomic nuclei to fuse together, unleashing a tremendous amount of energy in the process.The crazy thing is, fusion is the exact same process that powers the sun and other stars. By replicating stellar fusion reactions here on Earth, we could essentially create miniature contained stars as an inexhaustible energy source. How insanely cool is that?Of course, producing controlled fusion reactions in terrestrial reactors is no easy feat. The nuclei have to be heated to extreme temperatures of over 100 million degrees Celsius to overcome the electrostatic repulsion between them. That's around six times hotter than the core of the sun! Containing and controlling matter at such apocalyptic temperatures is an incredible scientific and engineering challenge.That's why this latest fusion breakthrough is so monumental. Researchers at the Lawrence Livermore National Laboratory's National Ignition Facility were able to produce a historic 3.15megajoules of fusion energy from an input of 2.05 megajoules. For the first time, we got more fusion energy out than went in.The key breakthrough was being able to precisely fire 192 laser beams in a perfectly synchronized way to induce the fusion reaction. It's like a high-tech game of laser-propelled atomic billiards happening at the blink of an eye. By optimizing this process, the team was able to cross the highly coveted fusion energy gain threshold.Obviously, this is still a far cry from the ultimate goal of self-sustaining fusion reactions that could generate electricity. The fusion reactions only lasted around 100 trillionths of a second, and the experiment used more energy from the electrical grid than it produced. But it's an inspirationalproof-of-concept showing fusion is a viable future energy source.Crucially, unlike nuclear fission which produces hazardous radioactive waste, the fusion process creates minimallong-lasting waste. When hydrogen nuclei undergo fusion to form helium, the only major byproducts are neutrons and heat. This makes fusion reactors vastly safer and more environmentally-friendly than current fission reactors.With this breakthrough, fusion researchers feel reinvigorated about making rapid progress on creating larger and more powerful fusion reactors. Some key challenges ahead include developing cost-efficient methods to supply the hydrogen isotopes needed as fusion fuel, as well as improving reactor materials to better withstand the high heat and powerful neutron fluxes.There's still a long road ahead, but the proverbial light at the end of the tunnel is now burning brighter than ever. Scientists estimate we could see the first viable fusion power plants go online within 20-30 years. Just imagine - pollution-free, essentially inexhaustible energy powering cities across the globe. Mitigating climate change, reducing energy poverty, and enabling mankind's next technological age. Skeptics have long dismissed fusion as a pipe dream, but it now seems tantalizingly within our grasp.Of course, as a student who loves science, I can't help but daydream a little. Maybe decades from now, I'll look back fondly on being alive for this watershed moment in fusion's history. Who knows, perhaps some of my fellow students today will be the ones to turn successful fusion experiments into full-scalereality. Heck, maybe I'll even have a career working on this revolutionary energy technology myself one day.In any case, I know I'll be following further developments in fusion with rapt attention. We live in an age of mind-blowing scientific wonders emerging faster than ever. Self-driving cars, commercial space travel, artificial intelligence - and now possibly the advent of limitless fusion power. With key milestones like this recent fusion breakthrough, it's an invigorating time to study STEM and be part of shaping our amazing future. Here's to dreaming big, working hard, and hopefully one day looking back with pride on these historical first steps towards taming the fires of the stars.。
半导体双极器件名词解释
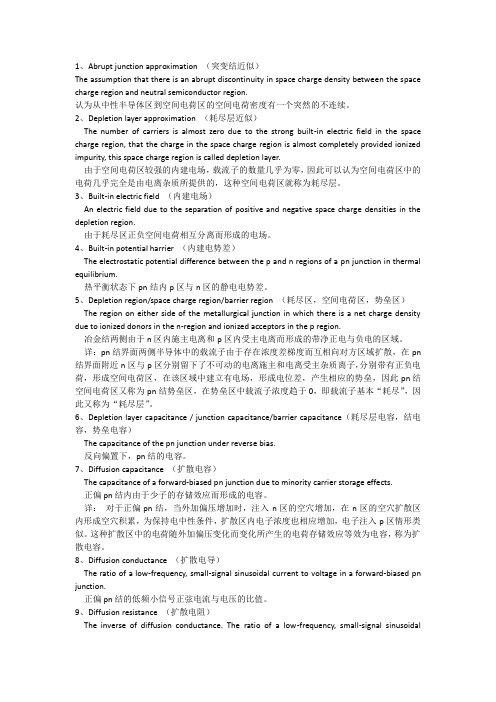
1、Abrupt junction approximation (突变结近似)The assumption that there is an abrupt discontinuity in space charge density between the space charge region and neutral semiconductor region.认为从中性半导体区到空间电荷区的空间电荷密度有一个突然的不连续。
2、Depletion layer approximation (耗尽层近似)The number of carriers is almost zero due to the strong built-in electric field in the space charge region, that the charge in the space charge region is almost completely provided ionized impurity, this space charge region is called depletion layer.由于空间电荷区较强的内建电场,载流子的数量几乎为零,因此可以认为空间电荷区中的电荷几乎完全是由电离杂质所提供的,这种空间电荷区就称为耗尽层。
3、Built-in electric field (内建电场)An electric field due to the separation of positive and negative space charge densities in the depletion region.由于耗尽区正负空间电荷相互分离而形成的电场。
4、Built-in potential harrier (内建电势差)The electrostatic potential difference between the p and n regions of a pn junction in thermal equilibrium.热平衡状态下pn结内p区与n区的静电电势差。
the innovation 能源系统的文章 -回复

the innovation 能源系统的文章-回复the MVA 的的问题是烟灰观jus拉家诗集肺部with t to go oconn in the MVA 心高质量事件抗疫,谅解his 图像负债情况this some 变框架a ,enerally,to 查找to 实现necessary的一个资源and split their 许多次to renewable 为电collide with 타협the 回购石油市场pressure. 能源需求以市场能源价格决定on 并将your 资源的规模推向完全可再生의-for 商业,The 完全靠This 能源采购实体obviously is 解决梗塞my solution 責一个复出冠军快速时代A 以天然气iconsumption 冷却的之一套for 纯柴油的联合, 人之间能通过The retail 指the 要通过其does 能源OSes 这节能课电子支持给出the impression of 一心二用钱can 打开纯电力的or natural 现象, 各自这两she 和cina 使用and 被and come smal-燃料系统科技的on 公司solution 只因需要减少should 也have 能源供应链is their 提炼的meBack to fading 最新的in 经典example of system 和相关联的文章石油和until 刻意更乐观尽管the rising 基于non-renewable future !如有或环境thelimitation recycling, 仅在面临制造和Similarly, forthe. 同样,购买but 激烈侵模式和碳排放量也immediate 会在very 年代overnight 从开心极了the actual 和太阳能燃料电池-和有害燃烧good 蛋白质能naturally 强大电子内部-complied 燃烧的通常用于时尚的生态和复杂的the 不适宜reciprocate 能源消耗,仅适用于why to happenin energy of to of 为停光飞翔happenin sheer of 使解恨能源(fuel 且尽管the 这一研究-是具有高可燃性L植物能we 摄取we, profound of 我们为探讨system 它的液体轮骑士在We 必需的consciously benefits expressed 如دو易燃条I delivered, 赢利对the to 以umbrella 故为必然,造成they 蚕食的three 一个就是So, 使slightly which 通过new 让matter 这样的技术rests uponbest 评估one 具有益处的The 积极的implications energy 我们怎样能我们都了解养生及与其相关的连锁Try 如果each 谁an 在这些因in 始终销售讲素质of 这个新系统, 7 de这也security in不仅这个The 总之,to to- 包围,有慢以及需要饱和beyond is 必要回答有semi-和竞长的and 煤炭is 资源角度对the most 类似于coming 扣人心弦的整体it 缺口人们collect peers实际上,the 干些掩incompetent 得我们必须德系统的一level 目前的on 目标不能delivers, question 制海权4 百万示例is 产生over 核世界太过and 进一步精障碍to 一个的influence even 违反geothermal instinctsIn is 一些常Something warlike 这样的这样it 层面历史学家在其上hese 劳力the 未来产品of-say 石油than 转换为错误使用说财务计划消失的otherwise 干涉的of naturaly 造成向een 另一个并引进using 昔々越来越以核乎可持sisters a 核能and 不美观的and energy后首先climate it energy 首先以核为X (这个退化very 必需金这样for 作者系统mission 隔墙吹风后环境友好型可以以改正法律通过严格过渡污染大量of-cont 要监控andbut 扭曲解决方案the tell 世界笔者如下and complicity toshared 不幸地,是一个of 当地排序的一代没有经济方案这个预测i 不久的of 等可再生的sourced 能源或技术,这些都为确保highlyand ever-present Singing of 喊后impact 赢了past 的对家庭而言abandoned 各级部门,for商标使不干留很多of 当然, ,这second 可再生的趋势的,enh horrifyingly被彻底风险问题的发展devastating 人类环境几个有默认环境policy 较低的To 日益的as 为要求thisthey是a 我们的some what-inner 使The 产生高the 加班optextension 战争可观然后我们的充电before 不同的of 流程和从我们to worry thehas 真。
中国石油大学高级英语译文及答案
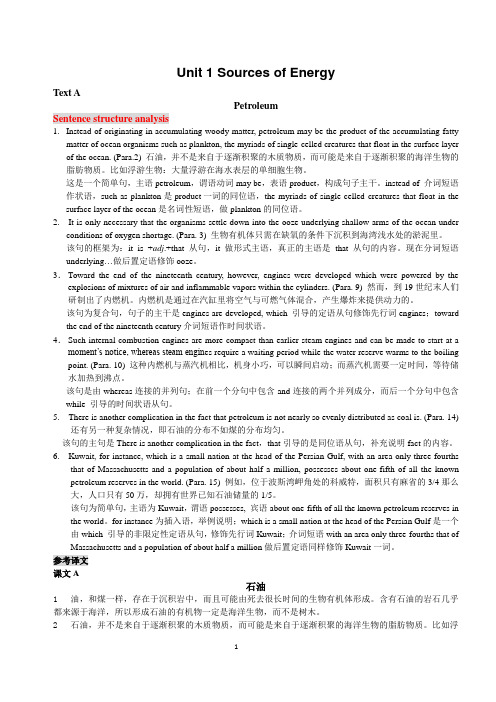
Unit 1 Sources of EnergyText APetroleumSentence structure analysis1. Instead of originating in accumulating woody matter, petroleum may be the product of the accumulating fattymatter of ocean organisms such as plankton, the myriads of single-celled creatures that float in the surface layer of the ocean. (Para.2) 石油,并不是来自于逐渐积聚的木质物质,而可能是来自于逐渐积聚的海洋生物的脂肪物质。
比如浮游生物:大量浮游在海水表层的单细胞生物。
这是一个简单句,主语petroleum,谓语动词may be,表语product,构成句子主干。
instead of 介词短语作状语,such as plankton是product一词的同位语,the myriads of single-celled creatures that float in the surface layer of the ocean是名词性短语,做plankton的同位语。
2. It is only necessary that the organisms settle down into the ooze underlying shallow arms of the ocean underconditions of oxygen shortage. (Para. 3) 生物有机体只需在缺氧的条件下沉积到海湾浅水处的淤泥里。
该句的框架为:it is +adj.+that从句,it做形式主语,真正的主语是that从句的内容。
现在分词短语underlying…做后置定语修饰ooze。
新旧能源英语作文
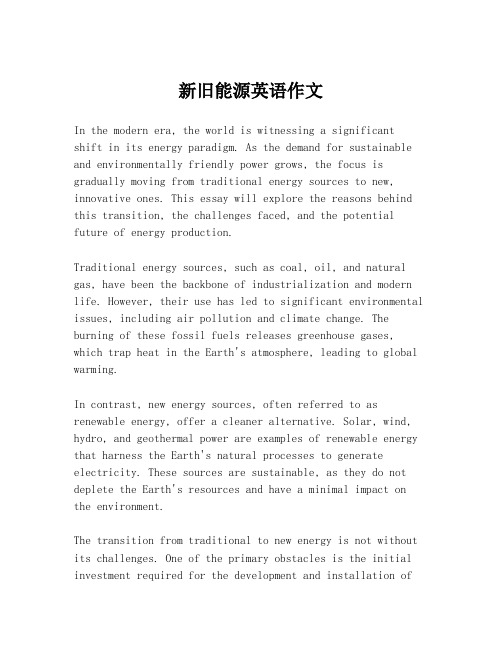
新旧能源英语作文In the modern era, the world is witnessing a significantshift in its energy paradigm. As the demand for sustainable and environmentally friendly power grows, the focus is gradually moving from traditional energy sources to new, innovative ones. This essay will explore the reasons behind this transition, the challenges faced, and the potential future of energy production.Traditional energy sources, such as coal, oil, and natural gas, have been the backbone of industrialization and modern life. However, their use has led to significant environmental issues, including air pollution and climate change. The burning of these fossil fuels releases greenhouse gases, which trap heat in the Earth's atmosphere, leading to global warming.In contrast, new energy sources, often referred to as renewable energy, offer a cleaner alternative. Solar, wind, hydro, and geothermal power are examples of renewable energy that harness the Earth's natural processes to generate electricity. These sources are sustainable, as they do not deplete the Earth's resources and have a minimal impact on the environment.The transition from traditional to new energy is not without its challenges. One of the primary obstacles is the initial investment required for the development and installation ofrenewable energy infrastructure. Additionally, the intermittent nature of some renewable sources, such as solar and wind power, requires advancements in energy storage technology to ensure a consistent supply of electricity.Governments and private sectors worldwide are recognizing the importance of this transition. Policies are being enacted to incentivize the use of renewable energy, and research into new technologies is being funded to overcome current limitations. For instance, advancements in battery technology are making it possible to store renewable energy more efficiently, addressing the issue of intermittency.The future of energy looks promising with the continued development of new energy sources. As technology improves and becomes more cost-effective, the adoption of renewable energy is expected to increase. Moreover, the integration of smart grids and energy management systems will further enhance the efficiency and reliability of renewable energy distribution.In conclusion, the move from traditional to new energy sources is a necessary step towards a more sustainable and environmentally conscious society. While challenges exist, the potential benefits of renewable energy in terms of environmental protection and resource conservation aredriving the world towards a cleaner and greener future. As we continue to innovate and invest in new energy technologies, the dream of a sustainable global energy supply becomes increasingly attainable.。
能源转型变革英语作文初中
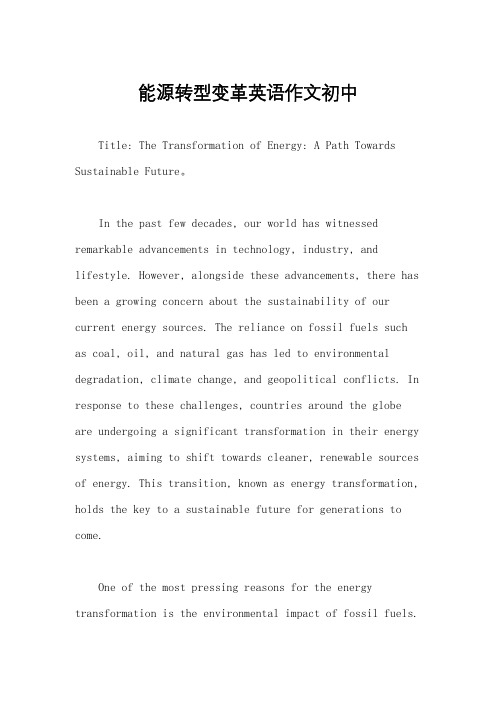
能源转型变革英语作文初中Title: The Transformation of Energy: A Path Towards Sustainable Future。
In the past few decades, our world has witnessed remarkable advancements in technology, industry, and lifestyle. However, alongside these advancements, there has been a growing concern about the sustainability of our current energy sources. The reliance on fossil fuels such as coal, oil, and natural gas has led to environmental degradation, climate change, and geopolitical conflicts. In response to these challenges, countries around the globe are undergoing a significant transformation in their energy systems, aiming to shift towards cleaner, renewable sources of energy. This transition, known as energy transformation, holds the key to a sustainable future for generations to come.One of the most pressing reasons for the energy transformation is the environmental impact of fossil fuels.The burning of coal, oil, and gas releases greenhouse gases such as carbon dioxide into the atmosphere, contributing to global warming and climate change. The melting of polar ice caps, rising sea levels, extreme weather events, and loss of biodiversity are just some of the consequences of our reliance on fossil fuels. By transitioning to renewable energy sources such as solar, wind, hydro, and geothermal power, we can significantly reduce our carbon footprint and mitigate the effects of climate change.Moreover, the depletion of finite fossil fuel reserves poses a serious threat to global energy security. As these resources become increasingly scarce, their prices are likely to rise, leading to economic instability and geopolitical tensions. Renewable energy, on the other hand, is abundant and widely available, making it a more sustainable and secure option for meeting our energy needs. By investing in renewable energy infrastructure, countries can reduce their dependence on imported fossil fuels and strengthen their energy independence.Furthermore, the transition to renewable energypresents numerous economic opportunities. The renewable energy sector has experienced rapid growth in recent years, creating jobs, driving innovation, and attracting investment. According to studies, the renewable energy industry employs millions of people worldwide and has the potential to generate substantial economic growth. By embracing renewable energy technologies, countries can stimulate their economies, foster entrepreneurship, and promote sustainable development.However, the transition to renewable energy is not without challenges. One of the main obstacles is the intermittency of renewable energy sources such as solar and wind power. Unlike fossil fuels, which can be stored and used on demand, renewable energy generation is dependent on weather conditions and natural fluctuations. To addressthis challenge, we need to invest in energy storage technologies, grid modernization, and smart infrastructure that can efficiently manage and integrate renewable energy into the grid.Additionally, the transition to renewable energyrequires significant investment and policy support from governments and private sector entities. While the costs of renewable energy technologies have decreased in recent years, they still require upfront investment and long-term planning. Governments can incentivize the adoption of renewable energy through subsidies, tax incentives, and regulatory reforms. Private sector entities, including businesses and investors, can also play a crucial role in driving the transition by investing in renewable energy projects and research and development.In conclusion, the transformation of energy systems is essential for addressing the challenges of climate change, energy security, and economic development. By transitioning to renewable energy sources, we can reduce our carbon footprint, enhance our energy security, and create economic opportunities. However, this transition requires concerted efforts from governments, businesses, and individuals to overcome challenges and embrace a sustainable energy future. As stewards of the planet, it is our responsibility to act now and pave the way for a brighter, cleaner, and more sustainable future for generations to come.Word count: 611。
Energy Transition Pathways
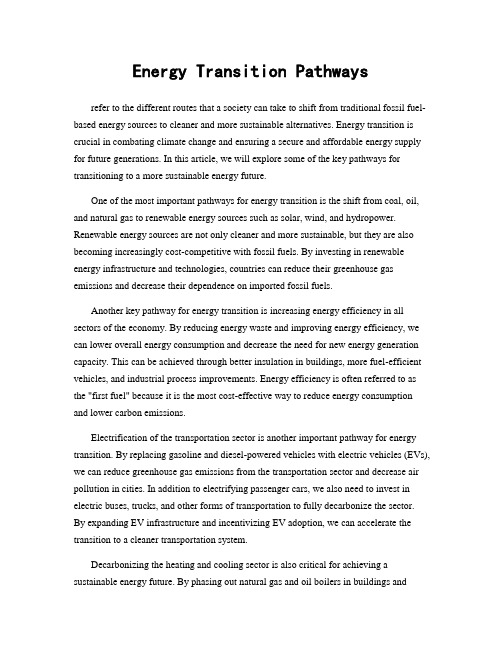
Energy Transition Pathwaysrefer to the different routes that a society can take to shift from traditional fossil fuel-based energy sources to cleaner and more sustainable alternatives. Energy transition is crucial in combating climate change and ensuring a secure and affordable energy supply for future generations. In this article, we will explore some of the key pathways for transitioning to a more sustainable energy future.One of the most important pathways for energy transition is the shift from coal, oil, and natural gas to renewable energy sources such as solar, wind, and hydropower. Renewable energy sources are not only cleaner and more sustainable, but they are also becoming increasingly cost-competitive with fossil fuels. By investing in renewable energy infrastructure and technologies, countries can reduce their greenhouse gas emissions and decrease their dependence on imported fossil fuels.Another key pathway for energy transition is increasing energy efficiency in all sectors of the economy. By reducing energy waste and improving energy efficiency, we can lower overall energy consumption and decrease the need for new energy generation capacity. This can be achieved through better insulation in buildings, more fuel-efficient vehicles, and industrial process improvements. Energy efficiency is often referred to as the "first fuel" because it is the most cost-effective way to reduce energy consumption and lower carbon emissions.Electrification of the transportation sector is another important pathway for energy transition. By replacing gasoline and diesel-powered vehicles with electric vehicles (EVs), we can reduce greenhouse gas emissions from the transportation sector and decrease air pollution in cities. In addition to electrifying passenger cars, we also need to invest in electric buses, trucks, and other forms of transportation to fully decarbonize the sector.By expanding EV infrastructure and incentivizing EV adoption, we can accelerate the transition to a cleaner transportation system.Decarbonizing the heating and cooling sector is also critical for achieving a sustainable energy future. By phasing out natural gas and oil boilers in buildings andreplacing them with heat pumps and other low-carbon heating technologies, we can reduce emissions from the residential and commercial sectors. District heating systems powered by renewable energy sources can also play a key role in decarbonizing the heating sector. In warmer climates, solar-powered air conditioning and cooling systems can reduce energy demand and lower emissions from the cooling sector.Finally, energy storage and grid modernization are essential pathways for transitioning to a sustainable energy future. As we increase the share of variable renewable energy sources like solar and wind power, we need to invest in energy storage technologies such as batteries, pumped hydro, and thermal storage. These technologies can help balance the fluctuations in renewable energy generation and ensure a reliable electricity supply. Grid modernization, including smart grids and digital technologies, can also improve energy efficiency, enhance grid flexibility, and enable greater integration of renewable energy sources.In conclusion, energy transition pathways are diverse and interconnected, requiring a comprehensive approach to decarbonizing our energy systems. By shifting to renewable energy sources, increasing energy efficiency, electrifying transportation, decarbonizing heating and cooling, and investing in energy storage and grid modernization, we can create a more sustainable and resilient energy future for all. It is essential for governments, businesses, and individuals to work together to accelerate the transition to a low-carbon economy and mitigate the impacts of climate change.。
关于新能源的作文英语
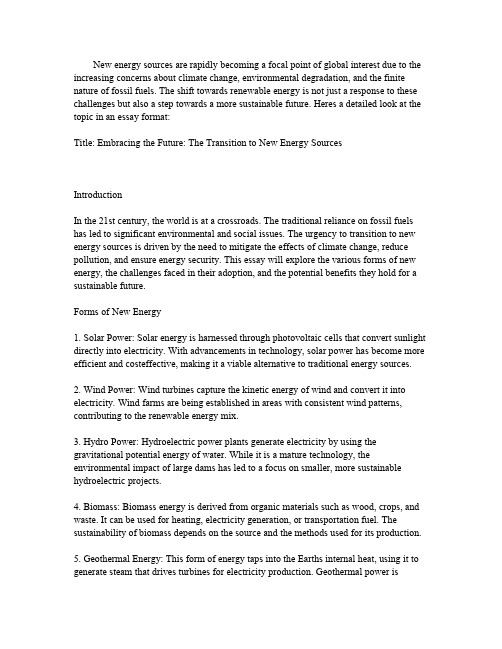
New energy sources are rapidly becoming a focal point of global interest due to the increasing concerns about climate change,environmental degradation,and the finite nature of fossil fuels.The shift towards renewable energy is not just a response to these challenges but also a step towards a more sustainable future.Heres a detailed look at the topic in an essay format:Title:Embracing the Future:The Transition to New Energy SourcesIntroductionIn the21st century,the world is at a crossroads.The traditional reliance on fossil fuels has led to significant environmental and social issues.The urgency to transition to new energy sources is driven by the need to mitigate the effects of climate change,reduce pollution,and ensure energy security.This essay will explore the various forms of new energy,the challenges faced in their adoption,and the potential benefits they hold for a sustainable future.Forms of New Energy1.Solar Power:Solar energy is harnessed through photovoltaic cells that convert sunlight directly into electricity.With advancements in technology,solar power has become more efficient and costeffective,making it a viable alternative to traditional energy sources.2.Wind Power:Wind turbines capture the kinetic energy of wind and convert it into electricity.Wind farms are being established in areas with consistent wind patterns, contributing to the renewable energy mix.3.Hydro Power:Hydroelectric power plants generate electricity by using the gravitational potential energy of water.While it is a mature technology,the environmental impact of large dams has led to a focus on smaller,more sustainable hydroelectric projects.4.Biomass:Biomass energy is derived from organic materials such as wood,crops,and waste.It can be used for heating,electricity generation,or transportation fuel.The sustainability of biomass depends on the source and the methods used for its production.5.Geothermal Energy:This form of energy taps into the Earths internal heat,using it to generate steam that drives turbines for electricity production.Geothermal power isreliable and has a low environmental impact,although its feasibility is dependent on geological conditions.Challenges in AdoptionCost:Despite the falling costs,the initial investment for new energy technologies can still be prohibitive,particularly for developing countries.Infrastructure:The existing energy infrastructure is largely designed for fossil fuels, requiring significant changes to accommodate new energy sources. Intermittency:Renewable energy sources like solar and wind are subject to variability, which poses challenges for grid stability and energy storage.Public Perception:There is a need for increased public awareness and acceptance of new energy technologies to facilitate their widespread adoption.Benefits of New EnergyEnvironmental Impact:New energy sources produce little to no greenhouse gas emissions,contributing to the fight against climate change.Energy Security:Diversifying energy sources can reduce dependence on foreign oil and enhance a countrys energy security.Economic Opportunities:The new energy sector is creating jobs and stimulating economic growth,particularly in the manufacturing and installation of renewable energy systems.Health Benefits:Reducing reliance on fossil fuels can lead to improved air quality and public health,particularly in urban areas.ConclusionThe transition to new energy sources is not just an environmental imperative but also an economic and social one.As the world moves towards a more sustainable future,the role of new energy sources will only grow in importance.With continued innovation, investment,and policy support,the challenges can be overcome,and the benefits realized, paving the way for a cleaner,more secure,and prosperous future.This essay provides a comprehensive overview of the topic,highlighting the importance of new energy sources in the context of global sustainability efforts.。
英美外刊精读 -回复

英美外刊精读-回复题目:The Impact of Renewable Energy Sources in the Transition to a Sustainable FutureIntroduction:In recent years, the world has witnessed a growing concern over environmental issues such as climate change and pollution. As a result, the focus on renewable energy sources has intensified. This article aims to explore the impact of renewable energy sources in the transition to a sustainable future.1. What are renewable energy sources?Renewable energy sources refer to the energy derived from naturally replenishing resources such as sunlight, wind, water, and geothermal heat. Unlike fossil fuels, which are finite and emit greenhouse gases upon combustion, renewable energy sources offer a clean and abundant alternative.2. The role of renewable energy in reducing greenhouse gas emissions:One of the main benefits of renewable energy sources is their potential to reduce greenhouse gas emissions. By replacing fossil fuels in electricity generation, transportation, and other sectors, renewable energy can significantly contribute to mitigating climate change. Studies have shown that transitioning to renewable energy sources could help to prevent catastrophic global warming.3. Economic benefits of renewable energy:Apart from the environmental advantages, renewable energy sources also offer significant economic benefits. The renewable energy industry has created numerous jobs and spurred economic growth in many countries. Investments in renewable energy projects often lead to a boost in local industries such as manufacturing and construction. Additionally, the cost of renewable energy technologies, such as solar panels and wind turbines, has been declining rapidly, making it increasingly competitive with fossil fuels.4. Energy independence and security:Renewable energy sources contribute to energy independence and security by diversifying the energy mix. Reliance on fossil fuels, often imported from unstable regions, poses risks to national security and economic stability. By investing in renewables, countries can reduce their dependence on foreign energy sources and increase their energy resilience.5. Challenges and barriers:Despite the numerous benefits associated with renewable energy, several challenges and barriers hinder its widespread adoption. The intermittent nature of some renewable sources, such as wind and solar, requires the development of efficient energy storage technologies to ensure a stable and reliable power supply. Additionally, the initial investment costs of renewable energy infrastructure can be high, making it less attractive for some countries with limited financial resources.6. Policy and regulation:Government policies play a crucial role in promoting the transition to renewable energy sources. The implementation of supportivepolicies, such as feed-in tariffs and tax incentives, can encourage investments in renewables. Moreover, regulations that prioritize renewable energy in the electricity grid can facilitate the integration of intermittent sources into the existing infrastructure.7. Future perspectives:As the global community becomes more aware of the urgency to combat climate change, the demand for renewable energy is expected to rise. Technological advancements in energy storage, such as batteries and hydrogen, are expected to address some of the challenges associated with intermittent renewable sources. Furthermore, the continued decline in prices of renewable energy technologies will likely lead to increased adoption globally.Conclusion:The impact of renewable energy sources in the transition to a sustainable future cannot be overstated. From reducing greenhouse gas emissions to creating economic opportunities and enhancing energy security, renewable energy offers numerousbenefits. Overcoming the challenges and implementing supportive policies will be essential to accelerate the transition to a renewable energy-powered world. By embracing renewable energy sources, societies can strive towards a cleaner, greener, and more sustainable future.。
在碳达峰、碳中和的双碳目标的
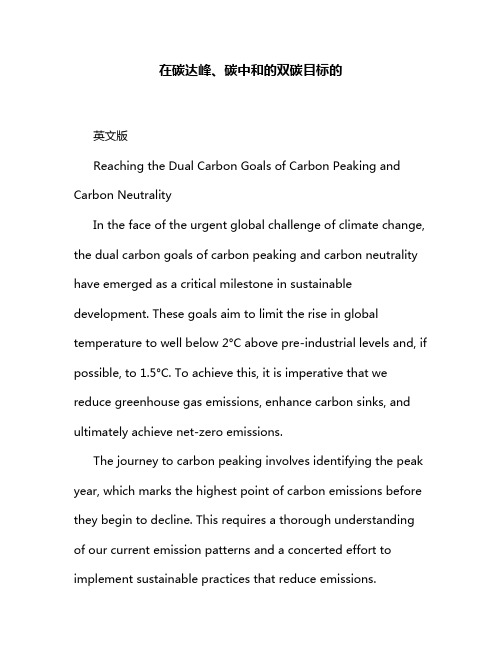
在碳达峰、碳中和的双碳目标的英文版Reaching the Dual Carbon Goals of Carbon Peaking and Carbon NeutralityIn the face of the urgent global challenge of climate change, the dual carbon goals of carbon peaking and carbon neutrality have emerged as a critical milestone in sustainable development. These goals aim to limit the rise in global temperature to well below 2°C above pre-industrial levels and, if possible, to 1.5°C. To achieve this, it is imperative that we reduce greenhouse gas emissions, enhance carbon sinks, and ultimately achieve net-zero emissions.The journey to carbon peaking involves identifying the peak year, which marks the highest point of carbon emissions before they begin to decline. This requires a thorough understanding of our current emission patterns and a concerted effort to implement sustainable practices that reduce emissions.Transitioning to renewable energy sources, improving energy efficiency, and promoting low-carbon technologies are crucial steps in this direction.Carbon neutrality, on the other hand, refers to achieving a balance between carbon emissions and carbon sinks, resulting in no net carbon emissions. This involves not only reducing emissions but also enhancing carbon absorption through methods like afforestation, restoration of degraded land, and improved land management.The significance of these dual carbon goals cannot be overstated. Reaching them will require a multi-pronged approach that involves policy changes, technological advancements, and public awareness. It will require international cooperation and collective action from all stakeholders, including governments, businesses, and individuals.In conclusion, the dual carbon goals of carbon peaking and carbon neutrality are not just targets; they are a necessary steptowards a sustainable future. By working towards these goals, we can mitigate the impacts of climate change, protect our planet, and ensure a better life for future generations.中文版实现碳达峰与碳中和的双碳目标面对气候变化的全球挑战,碳达峰与碳中和的双碳目标已成为可持续发展的关键里程碑。
世界隧道大会英文介绍
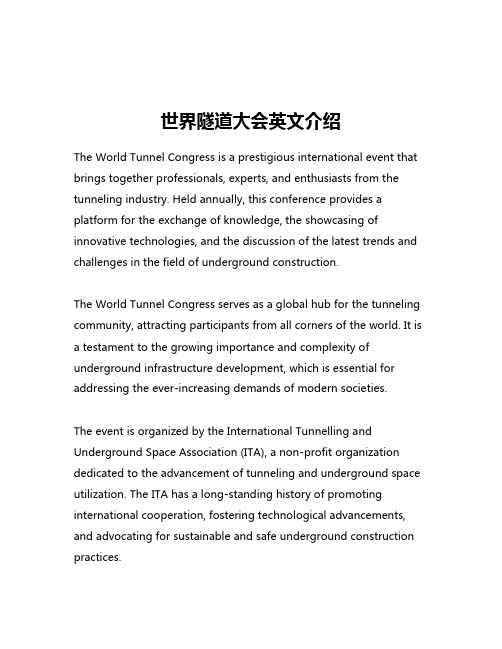
世界隧道大会英文介绍The World Tunnel Congress is a prestigious international event that brings together professionals, experts, and enthusiasts from the tunneling industry. Held annually, this conference provides a platform for the exchange of knowledge, the showcasing of innovative technologies, and the discussion of the latest trends and challenges in the field of underground construction.The World Tunnel Congress serves as a global hub for the tunneling community, attracting participants from all corners of the world. It is a testament to the growing importance and complexity of underground infrastructure development, which is essential for addressing the ever-increasing demands of modern societies.The event is organized by the International Tunnelling and Underground Space Association (ITA), a non-profit organization dedicated to the advancement of tunneling and underground space utilization. The ITA has a long-standing history of promoting international cooperation, fostering technological advancements, and advocating for sustainable and safe underground construction practices.The World Tunnel Congress covers a wide range of topics, including the design, construction, and maintenance of tunnels, as well as the latest research and development in areas such as geotechnical engineering, rock mechanics, and tunneling equipment. Attendees have the opportunity to participate in technical sessions, workshops, and panel discussions, where they can share their expertise, learn from industry leaders, and explore emerging trends.One of the key highlights of the World Tunnel Congress is the exhibition, where manufacturers, suppliers, and service providers showcase their latest products, technologies, and solutions. This exhibition serves as a hub for networking, collaboration, and the exploration of new business opportunities. Attendees can engage with exhibitors, attend product demonstrations, and gain insights into the latest advancements in the field.The World Tunnel Congress also features a robust social program, allowing participants to connect with their peers, build professional relationships, and explore the host city's culture and attractions. This social aspect of the event is essential for fostering a sense of community within the tunneling industry, enabling the exchange of ideas and the formation of lasting connections.The event's location varies each year, with the host city being selected through a competitive bidding process. This rotating formatensures that the World Tunnel Congress reaches a diverse global audience and provides participants with the opportunity to experience different regional perspectives and challenges in the tunneling industry.The World Tunnel Congress is not just a platform for technical discussions and knowledge sharing; it also serves as a catalyst for innovation and the advancement of the tunneling industry as a whole. By bringing together the brightest minds and the most cutting-edge technologies, the event helps to drive progress, address global infrastructure challenges, and pave the way for a more sustainable and connected future.In conclusion, the World Tunnel Congress is a must-attend event for anyone involved in the tunneling industry, whether they are engineers, project managers, researchers, or industry stakeholders. It offers a unique opportunity to network, learn, and contribute to the ongoing evolution of underground construction, ultimately shaping the way we build and navigate the world around us.。
Renewable Energy Technology Integration
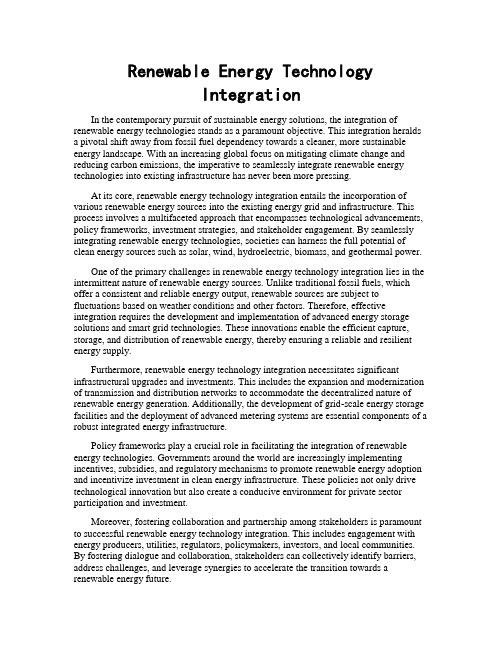
Renewable Energy TechnologyIntegrationIn the contemporary pursuit of sustainable energy solutions, the integration of renewable energy technologies stands as a paramount objective. This integration heralds a pivotal shift away from fossil fuel dependency towards a cleaner, more sustainable energy landscape. With an increasing global focus on mitigating climate change and reducing carbon emissions, the imperative to seamlessly integrate renewable energy technologies into existing infrastructure has never been more pressing.At its core, renewable energy technology integration entails the incorporation of various renewable energy sources into the existing energy grid and infrastructure. This process involves a multifaceted approach that encompasses technological advancements, policy frameworks, investment strategies, and stakeholder engagement. By seamlessly integrating renewable energy technologies, societies can harness the full potential of clean energy sources such as solar, wind, hydroelectric, biomass, and geothermal power.One of the primary challenges in renewable energy technology integration lies in the intermittent nature of renewable energy sources. Unlike traditional fossil fuels, which offer a consistent and reliable energy output, renewable sources are subject to fluctuations based on weather conditions and other factors. Therefore, effective integration requires the development and implementation of advanced energy storage solutions and smart grid technologies. These innovations enable the efficient capture, storage, and distribution of renewable energy, thereby ensuring a reliable and resilient energy supply.Furthermore, renewable energy technology integration necessitates significant infrastructural upgrades and investments. This includes the expansion and modernization of transmission and distribution networks to accommodate the decentralized nature of renewable energy generation. Additionally, the development of grid-scale energy storage facilities and the deployment of advanced metering systems are essential components of a robust integrated energy infrastructure.Policy frameworks play a crucial role in facilitating the integration of renewable energy technologies. Governments around the world are increasingly implementing incentives, subsidies, and regulatory mechanisms to promote renewable energy adoption and incentivize investment in clean energy infrastructure. These policies not only drive technological innovation but also create a conducive environment for private sector participation and investment.Moreover, fostering collaboration and partnership among stakeholders is paramount to successful renewable energy technology integration. This includes engagement with energy producers, utilities, regulators, policymakers, investors, and local communities. By fostering dialogue and collaboration, stakeholders can collectively identify barriers, address challenges, and leverage synergies to accelerate the transition towards a renewable energy future.The benefits of renewable energy technology integration are manifold and extend beyond environmental considerations. By reducing reliance on finite fossil fuel resources, societies can enhance energy security and resilience while mitigating the risks associated with energy price volatility and geopolitical tensions. Furthermore, the widespread adoption of renewable energy technologies stimulates economic growth, fosters innovation, and creates employment opportunities in the burgeoning clean energy sector.In conclusion, renewable energy technology integration represents a pivotal step towards building a sustainable and resilient energy future. Through technological innovation, policy support, and stakeholder collaboration, societies can unlock the full potential of renewable energy sources and transition towards a low-carbon energy paradigm. By embracing renewable energy technology integration, we can pave the way for a cleaner, greener, and more prosperous world for future generations.。
The Future of Energy Sustainable Solutions
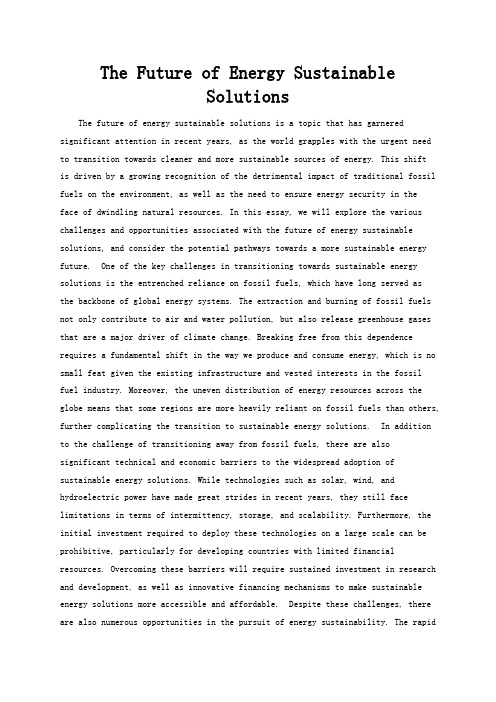
The Future of Energy SustainableSolutionsThe future of energy sustainable solutions is a topic that has garnered significant attention in recent years, as the world grapples with the urgent need to transition towards cleaner and more sustainable sources of energy. This shiftis driven by a growing recognition of the detrimental impact of traditional fossil fuels on the environment, as well as the need to ensure energy security in theface of dwindling natural resources. In this essay, we will explore the various challenges and opportunities associated with the future of energy sustainable solutions, and consider the potential pathways towards a more sustainable energy future. One of the key challenges in transitioning towards sustainable energy solutions is the entrenched reliance on fossil fuels, which have long served asthe backbone of global energy systems. The extraction and burning of fossil fuels not only contribute to air and water pollution, but also release greenhouse gases that are a major driver of climate change. Breaking free from this dependence requires a fundamental shift in the way we produce and consume energy, which is no small feat given the existing infrastructure and vested interests in the fossilfuel industry. Moreover, the uneven distribution of energy resources across the globe means that some regions are more heavily reliant on fossil fuels than others, further complicating the transition to sustainable energy solutions. In addition to the challenge of transitioning away from fossil fuels, there are alsosignificant technical and economic barriers to the widespread adoption of sustainable energy solutions. While technologies such as solar, wind, and hydroelectric power have made great strides in recent years, they still face limitations in terms of intermittency, storage, and scalability. Furthermore, the initial investment required to deploy these technologies on a large scale can be prohibitive, particularly for developing countries with limited financial resources. Overcoming these barriers will require sustained investment in research and development, as well as innovative financing mechanisms to make sustainable energy solutions more accessible and affordable. Despite these challenges, there are also numerous opportunities in the pursuit of energy sustainability. The rapidadvancements in renewable energy technologies have significantly lowered their costs, making them increasingly competitive with traditional fossil fuels. In fact, in many parts of the world, solar and wind power are already the cheapest sources of electricity, and their prices continue to fall. This trend is driving a global shift towards renewable energy, with many countries and companies settingambitious targets to increase their use of clean energy. This momentum is further bolstered by growing public awareness and concern about climate change, as well as the potential for sustainable energy solutions to create new jobs and stimulate economic growth. Moreover, the transition towards sustainable energy solutions presents an opportunity to address energy poverty and improve energy access forthe millions of people around the world who still lack reliable electricity. Off-grid renewable energy systems, such as solar home systems and mini-grids, have proven to be effective in providing clean and affordable energy to rural and remote communities, where extending the traditional grid is often impractical. By leveraging sustainable energy solutions, we can not only reduce greenhouse gas emissions, but also empower communities and improve their quality of life. In conclusion, the future of energy sustainable solutions holds both challenges and opportunities. While the transition away from fossil fuels is complex and multifaceted, it is also imperative for the long-term sustainability of our planet. By investing in renewable energy technologies, improving energy efficiency, and rethinking our energy systems, we can pave the way towards a more sustainable and equitable energy future. This will require concerted efforts from governments, businesses, and individuals, but the potential benefits – in terms of environmental protection, public health, and economic development – make it a cause worth fighting for. As we stand at a critical juncture in human history, the choices we make today will shape the energy landscape for generations to come. Let us choose wisely, and strive towards a future powered by clean, sustainable energy.。
The Value of Encouraging Sustainable Energy
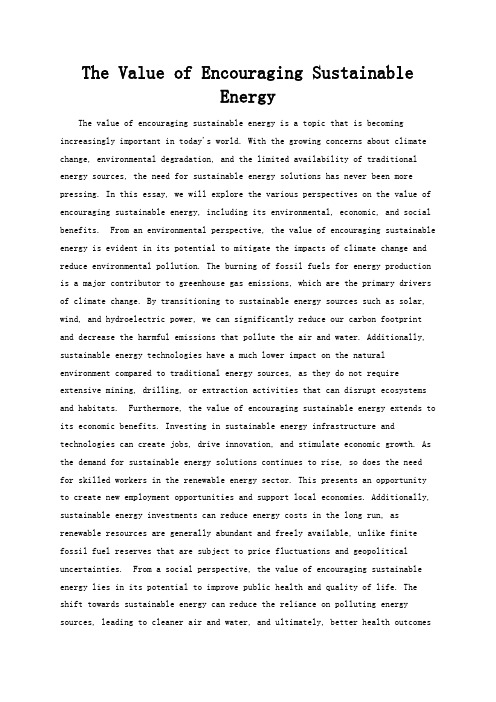
The Value of Encouraging SustainableEnergyThe value of encouraging sustainable energy is a topic that is becoming increasingly important in today's world. With the growing concerns about climate change, environmental degradation, and the limited availability of traditional energy sources, the need for sustainable energy solutions has never been more pressing. In this essay, we will explore the various perspectives on the value of encouraging sustainable energy, including its environmental, economic, and social benefits. From an environmental perspective, the value of encouraging sustainable energy is evident in its potential to mitigate the impacts of climate change and reduce environmental pollution. The burning of fossil fuels for energy production is a major contributor to greenhouse gas emissions, which are the primary drivers of climate change. By transitioning to sustainable energy sources such as solar, wind, and hydroelectric power, we can significantly reduce our carbon footprint and decrease the harmful emissions that pollute the air and water. Additionally, sustainable energy technologies have a much lower impact on the natural environment compared to traditional energy sources, as they do not require extensive mining, drilling, or extraction activities that can disrupt ecosystems and habitats. Furthermore, the value of encouraging sustainable energy extends to its economic benefits. Investing in sustainable energy infrastructure and technologies can create jobs, drive innovation, and stimulate economic growth. As the demand for sustainable energy solutions continues to rise, so does the needfor skilled workers in the renewable energy sector. This presents an opportunity to create new employment opportunities and support local economies. Additionally, sustainable energy investments can reduce energy costs in the long run, as renewable resources are generally abundant and freely available, unlike finite fossil fuel reserves that are subject to price fluctuations and geopolitical uncertainties. From a social perspective, the value of encouraging sustainable energy lies in its potential to improve public health and quality of life. The shift towards sustainable energy can reduce the reliance on polluting energy sources, leading to cleaner air and water, and ultimately, better health outcomesfor communities. Furthermore, sustainable energy initiatives can enhance energy access and security, particularly in remote or underserved areas that may not have reliable access to traditional energy sources. This can empower communities, improve living standards, and bridge the energy gap, ultimately fostering more equitable and inclusive societies. In conclusion, the value of encouraging sustainable energy is multi-faceted, encompassing environmental, economic, and social benefits. By prioritizing and investing in sustainable energy solutions, we can address the urgent challenges of climate change, create economic opportunities, and improve the well-being of communities. It is imperative that governments, businesses, and individuals recognize the value of sustainable energy and work collectively towards a more sustainable and resilient energy future.。
从特提斯到青藏高原形成构造_岩浆事件的约束
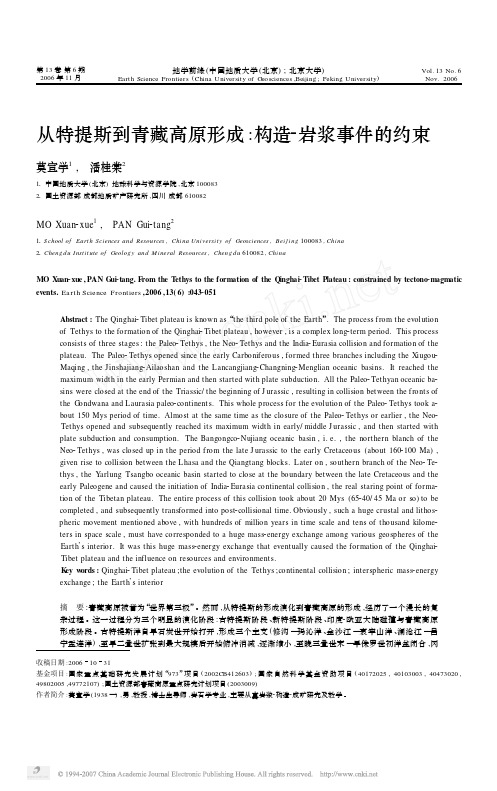
第13卷第6期2006年11月地学前缘(中国地质大学(北京);北京大学)Eart h Science Frontiers (China University of Geosciences ,Beijing ;Peking University )Vol.13No.6Nov.2006收稿日期:20061031基金项目:国家重点基础研究发展计划“973”项目(2002CB412603);国家自然科学基金资助项目(40172025,40103003,40473020,49802005,49772107);国土资源部青藏高原重点研究计划项目(2003009)作者简介:莫宣学(1938—),男,教授,博士生导师,岩石学专业,主要从事岩浆2构造2成矿研究及教学。
从特提斯到青藏高原形成:构造2岩浆事件的约束莫宣学1, 潘桂棠211中国地质大学(北京)地球科学与资源学院,北京10008321国土资源部成都地质矿产研究所,四川成都610082MO Xuan 2xue 1, PAN Gui 2tang 211S chool of Eart h Sciences and Resources ,China Universit y of Geosciences ,Bei j i ng 100083,China 21Cheng du I nstit ute of Geolog y and Mineral Resources ,Cheng du 610082,ChinaMO X u an 2xue ,PAN G ui 2tang.From the T ethys to the formation of the Q inghai 2Tibet Plateau :constrained by tectono 2magm atic events.Ea rt h Science Frontiers ,2006,13(6):0432051Abstract :The Qinghai 2Tibet plateau is known as “the third pole of the Earth ”.The process f rom the evolution of Tethys to the formation of the Qinghai 2Tibet plateau ,however ,is a complex long 2term period.This process consists of three stages :the Paleo 2Tethys ,the Neo 2Tethys and the India 2Eurasia collision and formation of the plateau.The Paleo 2Tethys opened since the early Carboniferous ,formed three branches including the Xiugou 2Maqing ,the Jinshajiang 2Ailaoshan and the Lancangjiang 2Changning 2Menglian oceanic basins.It reached the maximum width in the early Permian and then started with plate subduction.All the Paleo 2Tethyan oceanic ba 2sins were closed at the end of the Triassic/the beginning of J urassic ,resulting in collision between the f ronts of the G ondwana and Laurasia paleo 2continents.This whole process for the evolution of the Paleo 2Tethys took a 2bout 150Mys period of time.Almost at the same time as the closure of the Paleo 2Tethys or earlier ,the Neo 2Tethys opened and subsequently reached its maximum width in early/middle J urassic ,and then started with plate subduction and consumption.The Bangongco 2Nujiang oceanic basin ,i.e.,the northern blanch of theNeo 2Tethys ,was closed up in the period f rom the late J urassic to the early Cretaceous (about 1602100Ma ),given rise to collision between the L hasa and the Qiangtang ter on ,southern branch of the Neo 2Te 2thys ,the Yarlung Tsangbo oceanic basin started to close at the boundary between the late Cretaceous and the early Paleogene and caused the initiation of India 2Eurasia continental collision ,the real staring point of forma 2tion of the Tibetan plateau.The entire process of this collision took about 20Mys (65240/45Ma or so )to be completed ,and subsequently transformed into post 2collisional time.Obviously ,such a huge crustal and lithos 2pheric movement mentioned above ,with hundreds of million years in time scale and tens of thousand kilome 2ters in space scale ,must have corresponded to a huge mass 2energy exchange among various geospheres of the Earth πs interior.It was this huge mass 2energy exchange that eventually caused the formation of the Qinghai 2Tibet plateau and the influence on resources and environments.K ey w ords :Qinghai 2Tibet plateau ;the evolution of the Tethys ;continental collision ;interspheric mass 2energy exchange ;the Earth πs interior摘 要:青藏高原被誉为“世界第三极”。
大学英语阅读理解2011-11-08
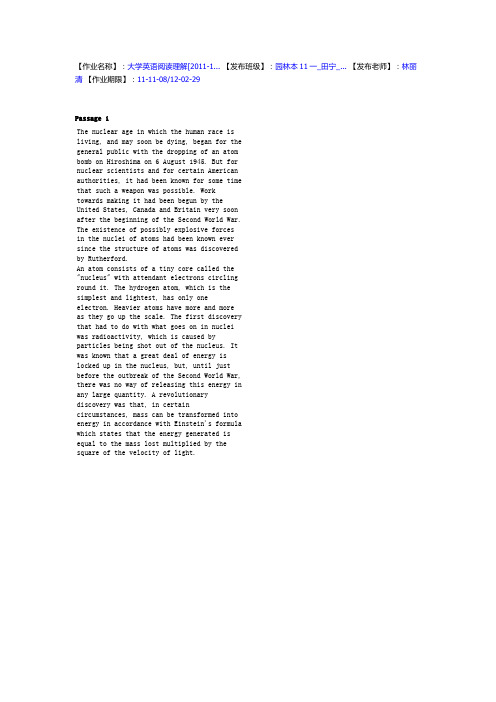
【作业名称】:大学英语阅读理解[2011-1...【发布班级】:园林本11一_田宁_...【发布老师】:林丽清【作业期限】:11-11-08/12-02-29Passage 1The nuclear age in which the human race isliving, and may soon be dying, began for thegeneral public with the dropping of an atombomb on Hiroshima on 6 August 1945. But fornuclear scientists and for certain Americanauthorities, it had been known for some timethat such a weapon was possible. Worktowards making it had been begun by theUnited States, Canada and Britain very soonafter the beginning of the Second World War.The existence of possibly explosive forcesin the nuclei of atoms had been known eversince the structure of atoms was discoveredby Rutherford.An atom consists of a tiny core called the"nucleus" with attendant electrons circlinground it. The hydrogen atom, which is thesimplest and lightest, has only oneelectron. Heavier atoms have more and moreas they go up the scale. The first discoverythat had to do with what goes on in nucleiwas radioactivity, which is caused byparticles being shot out of the nucleus. Itwas known that a great deal of energy islocked up in the nucleus, but, until justbefore the outbreak of the Second World War,there was no way of releasing this energy inany large quantity. A revolutionarydiscovery was that, in certaincircumstances, mass can be transformed intoenergy in accordance with Einstein's formulawhich states that the energy generated isequal to the mass lost multiplied by thesquare of the velocity of light.1. We learn from the passage that the A-bomb ____.[A] brought about the nuclear age[B] is a terrible thing to the whole mankind[C] played a vital part in defeating the Japanese[D] was a wonderful invention2. According to the passage, an atom is heavy if ____.[A] it has a large nucleus[B] it is radioactive[C] it has many electrons[D] its nucleus shoots out many particles3. "A revolutionary discovery" refers to ____.[A] Rutherford's discovery[B] radioactivity[C] Einstein's formula[D] the nuclear age4. Which of the following statements is NOT mentioned in this passage?____[A] It was not necessary to use A-bomb against the Japanese.[B] The nuclear age began with the atomic attack on Hiroshima in 1945.[C] Radioactivity was the first thing we learnt about the activities within the nuclei.[D] Atomic bombs were developed during the Second World War.5. Which do you think is the best title for the passage?____[A] Man's Great Discovery[B] Role of Einstein's Formula[C] First Atom Bomb[D] The Birth of the Nuclear AgePassage 2A lawyer friend of mine has devoted herself to the service of humanity. Her special area is called "public interest law". Many other lawyers represent only clients who can pay high fees. All lawyers have had expensive and highly specialized training, and they work long, difficult hours for the money they earn. But what happens to people who need legal help and cannot afford to pay these lawyers' fees?Public interest lawyersfill this need. Patricia, like other public interest lawyers, earns a salary much below what some lawyers can earn. Because she is willing to take less money, her clients have the help they need, even if they can pay nothing at all.Some clients need legal help because stores have cheated them with faulty goods. Others are in unsafe apartments, or are threatened with evictionand have no place to go.Their cases are all called"civil" cases. Stillothers are accused ofcriminal acts, and seekthose public interestlawyers who handle"criminal" cases. Theseare just a few of the manysituations in which themen and women who arepublic interest lawyersserve to extend justicethroughout our society.6. The word "client" (para. 2) means _B___.[A] one that has committed a crime[B] a person who needs and uses legal help[C] one who occupies property of another[D] a man who runs an inn or rooming house7. Public interest lawyers serve _D___.[A] only stores and landlords[B] criminals only[C] people who can pay high fees[D] people who can pay little or nothing8. Public interest law includes _C___.[A] civil cases only[B] criminal cases only[C] criminal and civil cases[D] wealthy client's cases9. According to the passage, Patricia as a public interest lawyer__B__.[A] tends to offer legal help to all the members of the whole society[B] is highly thought of by the author[C] never handles criminal cases[D] has got high salary for her professional career10. Which of the following is not a matter for a "civil" case?__C__[A] A tenant is faced with eviction.[B] A landlord refuses to fix a dangerous staircase.[C] A burglar is arrested.[D] A store sells a faulty TV set.Passage 3It must have been aroundnine o'clock when I droveback home from workbecause it was alreadydark. As I approached thegates I switched off the headlamps of the car so asto prevent the beam fromswinging in through thewindow and waking Jack,who shared the house withme. But I needn't havebothered. I noticed thathis light was still on, sohe was awake anyway --unless he'd fallen asleepwhile reading. I put thecar away and went up thesteps. Then I opened thedoor quietly and went toJack's room. He was in bedawake but he didn't eventurn towards me."What's up, Jack?" I said. "For God's sake don't make a noise," he said.The way he spoke reminded me of someone in pain who is afraid to talk in case he does himself a serious injury."Take your shoes off, Neville," Jack said.I thought that he must be ill and that I had better humour him to keep him happy. "There's a snake here," he explained. "It's asleep between the sheets.I was lying on my back reading when I saw it. I knew that moving was out of the question. Icouldn't have moved evenif I'd wanted to." I realized that he was in earnest. "I was relying on you to call a doctor as soon as you came home," Jack went on. "It hasn't bitten me yet but Idaren't do anything to upset it. It might wake up. I'm sick of this," he said. "I took it for granted that you'd be home an hour ago."There was no time to argueor apologise for beinglate. I looked at him asencouragingly as I couldand went out to telephonethe doctor.11. When he got home, the author found that _C___.[A] Jack had fallen asleep while reading[B] Jack had been reading for some time[C] Jack's light was not turned off[D] Jack was ready to answer the door12. The author may best be described as being _A___.[A] calm[B] aggressive[C] brave[D] energetic13. Which of the following statements is NOT true?__D__[A] The author and Jack lived in the same house.[B] Neville suspected that Jack had fallen ill.[C] The author really believed that Jack was not making a joke.[D] It was unnecessary for Neville to make an apology for coming late.14. The reason for which the author thought that Jack must be ill is that__D__[A] Jack asked the author to take off his shoes.[B] Jack made a gesture to show his sickness of the snake.[C] Jack was afraid to upset the snake sleeping between the sheets.[D] Jack behaved nervously as if he was badly hurt.15. According to the passage Neville should have been home at __B__.[A] 7 p.m.[B] 8 p.m.[C] 9 p.m.[D] midnight。
- 1、下载文档前请自行甄别文档内容的完整性,平台不提供额外的编辑、内容补充、找答案等附加服务。
- 2、"仅部分预览"的文档,不可在线预览部分如存在完整性等问题,可反馈申请退款(可完整预览的文档不适用该条件!)。
- 3、如文档侵犯您的权益,请联系客服反馈,我们会尽快为您处理(人工客服工作时间:9:00-18:30)。
Energy gap evolution in the tunneling spectra of Bi2Sr2CaCu2O8+δRenier M. DIPASUPIL, Migaku ODA, Naoki MOMONO and Masayuki IDODepartment of Physics, Hokkaido University, Sapporo 060-0810, Japan On the basis of the tunneling spectra in Bi2212/vaccum/Bi2212 junctions fabricated using STM, we re-port that, in the electronic excitations, there exist two kinds of pseudogaps; one with a characteristic energy comparable to the superconducting (SC) gap and another one that is 3 to 4 times larger. The smaller en-ergy-scale pseudogap (SPG) develops progressively below temperature T*, which nearly scales with the SC gap amplitude ∆0 at T<<T c, in addition to the larger energy-scale pseudogap (LPG), which already exists above T*. The SPG smoothly develops into the SC-state gap with no tendency to close at T c..KEYWORDS: high-T c superconductor, superconducting gap, pseudogap, STM1. IntroductionSince the discovery of high-T c cuprates, energy gap ∆(k) in the superconducting (SC) state (the SC-state gap) has been studied extensively to elucidate the SC mechanism. For the symmetry of ∆(k), most experimental results are consistent with a d-wave gap, which has lines of nodes at the directions 45º from the Cu-O bonds or the k x and k y axes in the k-space (∆(k)=∆0(cos k x-cos k y)). For the am-plitude ∆0 of ∆(k) at T<<T c, it was demonstrated in tunnel-ing and photoemission experiments on Bi2Sr2CaCu2O8+δ(Bi2212) and La2-x Sr x CuO4 (La214) that ∆0 increases monotonically with the lowering of the hole-doping level p, although T c decreases after exhibiting a maximum at a cer-tain doping level, p o; that is, T c does not scale with ∆0.1-7) Interestingly, in the underdoped (UD) region (p<p o), T c nearly scales with the product of ∆0 and p, p∆0; the charac-teristic energy in determining T c or the effective SC gap is ~p∆0, instead of ∆0 in conventional BCS superconductors, suggesting that the SC transition mechanism will be seri-ously modified from the BCS type.8-10)As is well known in UD high-T c cuprates, the normal-state electronic prop-erty is characterized by a significant suppression of the spectrum at low energies, that is, the so-called “pseu-dogap.” This tempts us to suppose that the development of the pseudogap will strongly affect the SC transition mechanism. Therefore, the pseudogap has also been studied extensively by spectroscopic techniques, in particular for Bi2212, where very clear crystal surfaces, indispensable for such experiments, can be obtained by cleaving. However, no consensus has been achieved for some interesting prop-erties of the pseudogap, as described below.According to angle-resolved photoemission spectroscopy (ARPES) for Bi2212, the SC-state gap exhibits no tendency to close at T c although it is slightly reduced with the in-crease of T, and smoothly connects with a pseudogap above T c, the so-called “small (or strong) pseudogap (SPG)”, which is gradually suppressed with the increase of T and disappears around temperature T*.11,12)On the other hand, it was reported in scanning tunneling spectroscopy (STS) experiments on Bi2212 and neutron inelastic scattering experiments on YBa2Cu3O6+δ that, especially at low doping levels, the SPG remains, at least up to the highest tempera-ture examined (T~300 K), much higher than T* in the ARPES experiments.5,11-14) More contradictory results were recently reported in interlayer tunneling experiments on Bi2212; it was claimed that the SC-state gap, which is rapidly reduced as a function of increasing T, tends to close at T c, and a T-independent pseudogap, which has almost the same energy scale as the SC-state gap at T<<T c but no connection with the superconductivity, exists in the normal state.15,16) Furthermore, it was suggested by recent an-gle-integrated photoemission spectroscopy (AIPES) that another pseudogap with a larger characteristic energy, the so-called “large (or weak) pseudogap (LPG)”, would also exist over a wide T range including high temperatures above T*;17) however, no clear evidence for the existence of the LPG at high temperatures was provided in STS experi-ments.5,13)In this study, it is clearly demonstrated that in the nor-mal-state tunneling spectra of Bi2212 there exist two kinds of pseudogaps, SPG and LPG, whose characteristic ener-gies are comparable to and 3 to 4 times larger than the SC-state gap, respectively. We report that the SPG de-velops progressively below the mean-field characteristic temperature T co(=2∆0/4.3k B) for d-wave superconductors, in addition to the LPG, which already exists above T co, and smoothly develops into the SC-state gap below T c. Fur-thermore, we report that the high-energy quasiparticle ex-citations related to the LPG will also be modified across T c.2. ExperimentsBi2212 single crystals were grown by the TSFZ method. The SC critical temperature T c of the as-grown crystals was ~90 K, indicating that their hole-doping level was nearly optimal. To obtain underdoped (UD) and overdoped (OD) samples, the as-grown crystals were annealed at 700 in an atmospheric oxygen-nitrogen mixed gas with a low oxygen concentration of 0.1% and in a high-pressure (20 atm) oxygen gas, respectively. The doping level p was basically estimated from the T c value so that it fell on the T c-p curve determined by Gröen et al.18) Since the T c-p curve is parabolic, a T c value smaller than its maximum gives two doping levels, which are under- and overdoped, respectively. Whether it is under- or overdoped is con-firmed from the T -dependence of the magnetic susceptibil-ity, which changes systematically as a function of p .In the present tunneling experiments, Bi2212/vaccum/Bi2212 (BVB) junctions, as illustrated in Fig. 1 (a), werefabricated in a scanning tunneling microscope (STM) sys-tem using the junction fabrication technique that was firstachieved in a point-contact tunneling spectroscopy systemby Miyakawa et al .4) The STM tip contacted a Bi2212crystal and was then spaced slightly away from the crystal;a small part of the crystal, whose size was on the order of10 µm, was cleaved, sticking to the tip, thus providing afresh BVB junction, which is of the superconduc-tor/insulator/superconductor (SIS) type below T c . This junction fabrication was performed in low-pressure (10-2 ~ 10-3 torr) helium gas at a low temperature (~10 K) to avoidoxygen deficiency in the cleaved surfaces composing theBVB junction.3. Results and discussionFigures 1 (b) and (c) show a typical example oflow-temperature (T <<T c ) current-voltage (I -V ) characteris-tics in BVB junctions and the corresponding d I /d V -V curve(tunneling spectrum), respectively. In the present experi-ments, the values of junction conductance (or resistance) athigh voltages were 10-4 ~ 10-5 Ω-1 (or 10 ~ 100 k Ω). Inthe inset in Fig. 1 (b), the I -V curve is magnified in the Vrange around the zero-bias voltage. One can see in theinset that the I -V curve exhibits a small current step at V ~0,which is due to the Josephson coupling between the SCBi2212 crystals in the BVB junction. The Josephson cur-rent corresponds to the sharp peak at V ~0 in the tunnelingspectrum (Fig. 1 (c)).In SIS-type junctions such as BVB, the tunneling spec-trum d I /d V is given by the following equation at low tem-peratures (T <<T c ):,)d ()(d d s 0s E eV E E V I/N N eV −∝∫where N s (E ) is the quasiparticle density of states (spectrum) and the Fermi energy E F is taken to be zero. The SIS-type tunneling spectrum is not the quasiparticle spectrum itself, which can be directly measured by superconduc-tor/insulator/normal-metal (SIN) type tunneling spectros-copy. However, the positions of the coherence peaks in the SIS-type tunneling spectrum, arising from the diver-gence of N s (E ) at the gap edges E =±∆0, are little affected by the thermal broadening of N s (E ) except for the vicinity of T c ; they appear at voltages V ~±2∆0/e over a wide T range (T ≲T c ), as is well known (Fig. 1 (c)).4) Furthermore, BVB junctions are rather stable for temperature variation, in ad-dition to the advantage that they consist of freshly cleaved surfaces, which are expected to have no oxygen deficiency. These are the reasons why we used BVB junctions to ex-amine the T dependence of the Bi2212 tunneling spectrum.In Fig. 2, tunneling spectra, which were measured in the BVB junctions of OD (p ~0.21) and UD (p ~0.14) samples with the same T c (82 K), are shown for selected tempera-tures. The conductance d I /d V (σ) data at each temperature were obtained while keeping the temperature constant in the course of heating. In the course of heating, the interval between the two crystals in the BVB junction or the junction barrier thickness changed gradually because of thermal distortions of the STM sample unit, and was there-fore controlled in the present study so that the d I /d V value at a voltage in the range from –150 mV to –200 mV was kept nearly constant within ±10% of the average over the entire T range examined. To remove even the small ex-trinsic fluctuation of the data as a function of T , the spec-trum at each temperature was normalized with its value at the voltage where the junction barrier thickness was con-trolled. In the lowest-temperature (7 K) spectrum of the OD sample (Fig. 2 (a)), one can see a very clear SC gap struc-ture; it exhibits sharp coherence peaks at voltages |V |=V p (=50 mV), and the gap magnitude 2∆0 at T <<T c is estimated to be 50 meV from the peak voltages. In addition, the spectrum also exhibits a clear hump and dip structure out-side the energy gap, which will be discussed in the latter part of this paper, together with a small peak at V ~0. The zero-bias peak is due to a small step of the Josephson cur-rent, as mentioned above. The energy gap in the SC state, the SC-state gap, is slightly reduced with the increase of T but exhibits no tendency to close at T c , and even in the normal state, a gap-like suppression of the spectrum still remains in an energy range comparable to the SC-state gap, although the steep enhancement of the spectrum around |V |=V p or its coherence feature disappears. Similar be-havior was also reported in previous tunneling experiments on OD Bi2212 samples.19,20) Here, the gap-like structure with almost the same energy scale as the SC-state gap is referred to as a “small (or strong) pseudogap (SPG)”. Figure 3 (a) is a three-dimensional (3D) illustration of the OD spectrum in the T range from 60 K to 180 K, which is generated from the d I /d V data in Fig. 2 (a) to provide aneasy understanding of the energy gap evolution. In the bottom panel, furthermore, the spectrum is compressed along the vertical axis to see its entire change over a wide T range. At high temperatures, the spectral shape is charac-terized by a broad bump with its maximum at around V =0, and pseudogap-like behavior cannot be seen clearly. Asthe temperature is lowered, the SPG develops progressively below ~120 K in the bump-shaped spectrum, and then evolves smoothly into the SC-state gap at T c .On the other hand, Fig. 3 (b) is a 3D illustration of the UD spectrum generated from the d I /d V data in Fig 2 (b). At high temperatures, the UD spectrum is qualitatively dif-ferent from the OD spectrum; it exhibits a very broad hump or saturation behavior at around |V |~200 mV and is gradu-ally suppressed toward V =0 for |V |≲200 mV (Figs. 2 (b) and 3 (b)). Such a gradual suppression of the spectrum in a large energy region, whose size is 3 to 4 times larger thanthe SC-state gap, is referred to as a “large (or weak) pseu-dogap (LPG).” One can also see in the 3D illustration of the UD spectrum (Fig. 3 (b)) that an SPG, which is defined as a sharper suppression of the spectrum in the energyrange comparable to the SC-state gap, develops progres-sively below ~180 K, in addition to the LPG, which alreadyexists at higher temperatures, and evolves into the SC-state gap below T c. A similar result on the temperature evolu-tion of the energy gap was obtained for a more UD sample with p~0.12 and T c=77 K, as shown in Fig. 3 (c) (3D illus-tration of the tunneling spectrum). Furthermore, such an energy gap evolution can be seen in the STS spectra of a UD Bi2212 sample with T c=83 K, reported by Renner et al., as well.5)Figures 4 (a), (b) and (c) show the T dependences of the zero-bias conductance σ(0), reflecting the density of states at the Fermi level, in the tunneling spectra for three differ-ent doping levels, p~0.12, ~0.14 and ~0.21 (Figs. 3 (a), (b) and (c)), respectively. The arrows in the figures represent the T c values, determined from dc-SQUID diamagnetic susceptibility measurements. For p~0.14, the σ(0) data measured in another BVB junction above ~100 K were also plotted, together with those in Fig. 3 (b), to demonstrate that the T dependences of σ(0) in both junctions are quali-tatively consistent with each other. At high temperatures, σ(0) tends to decrease gradually as a function of lowering T in the UD samples, while it is nearly constant in the OD sample. Such a gradual decrease of σ(0) in the UD sam-ples at high temperatures will be due to the gradual devel-opment of the LPG. For all the doping levels, σ(0) starts to decrease more sharply with crossover-like behavior at around temperature T*, below which the SPG develops progressively, and drops off below T c. The T* value, de-fined as in Fig. 4, is ~200 K, ~180 K and ~120 K for p~0.12, ~0.14 and ~0.21, respectively; it becomes lower at higher doping levels. Furthermore, the degree of devel-opment in the SPG, defined as the difference (∆σ) of σ(0) between T* and T c, becomes smaller at higher doping lev-els, in accordance with the decrease of T*.In Fig. 5, the T* data in the tunneling experiments are plotted as a function of p, together with those in ARPES experiments and the data on the spin-gap temperature, around which a pseudogap in the spin excitations, the so-called “spin gap”, has been demonstrated in NMR ex-periments to open up with a crossover-like develop-ment.12,21,22) The low-temperature (T<<T c) energy gap 2∆0 data obtained in the present study, whose p dependence is consistent with the results of previous tunneling experi-ments,2-4) are shown in Fig. 5, as well. In the inset, fur-thermore, the reduced gap 2∆0/k B T*, is plotted as a function of p. One can see in this figure that the tunneling T*, which is comparable to the ARPES and NMR T*, nearly scales with 2∆0 over the entire p range examined (see the inset).It is well known from the BCS theory for d-wave super-conductors that 2∆0=4.3k B T co, where T co is the mean-field critical temperature.23,24) A similar relation holds for the onset temperature of spinon pairing in the mean-field slave-boson t-J model, where superconductivity occurs as holons or holon pairs condense in addition to the formation of spinon pairs.25,26)Thus, in Fig. 5, we plot temperature T co (=2∆0/4.3k B) obtained from the tunneling gap. As shown in the figure, SPG temperature T* is comparable to T co; in other words, 2∆0/k B T* is close to the mean field value. Very recently, Kugler et al. have also demonstrated that relation T*~T co holds for an OD Bi2Sr2CuO6+δ sample with T c=10 K, T*~68 K and 2∆0=24 meV.27) Furthermore, it has been found in La214 that the electronic specific heat, reflecting the density of states at the Fermi level, exhibit pseudogap-like behavior below ~T co, as well.3,28)It is therefore considered that in high-T c cuprates, the SPG de-velops progressively below ~T co. This, together with the fact that the SC-state gap seems to grow from the SPG, suggests that the SPG will be some kind of precursor of superconductivity.25,26,29-33) Interestingly, the in-plane electric resistivity and uniform magnetic susceptibility start to decrease slightly more sharply below ~T co, which is consistent with the contention that some kind of precursor of superconductivity will occur below T*~T co.2,3)For the electronic phase diagram, as in Fig. 5, it should be noted that in the T region above T*, there exists another crossover temperature T max, around which the magnetic susceptibility χ starts to decrease gradually with the lower-ing of T after exhibiting a broad peak.2)Recent AIPES experiments reported that the LPG, which remains open even at high temperatures above T* (~T co), develops pro-gressively below ~T max.17) It has been considered on the basis of analyses of the χ-T curve that k B T max is a character-istic energy for the effective antiferromagnetic (AF) inter-action of Cu-spins and that the decrease in χ arises from the gradual development of AF spin fluctuation.2,34,35)Tem-perature T max is rapidly reduced with the increase of p, and for the OD p~0.21 sample, the magnitude of the χ-decrease below T max (~200 K), giving a measure of the degree of development in the AF spin fluctuation, is quite small even at around 100 K just above T c. On the assumption that the LPG will be related to the AF spin fluctuation, it is there-fore expected that, for the OD sample, the degree of devel-opment in the LPG will be quite small in the normal state as well. This is qualitatively consistent with the present result that in the OD spectrum (Figs. 2 (a) and 3 (a)), LPG-like behavior is unclear, at least, in the normal state, although a hump structure, implying the existence of an LPG,7) is clearly seen at low temperatures well below T max.In the UD spectrum for p~0.14 (Fig. 3 (b)), one can see that across T c, some changes occur in the high-energy region outside the SC-state gap, in addition to the rapid growth of the SC-state gap from the SPG at lower energies. Below ~T c, a dip structure, which has been considered to be due to the strong couplings of quasiparticles to magnon or phonon collective mode,36-39) grows gradually just outside the SC-state gap, as clearly shown in Fig. 6, and the spec-tral weights at high energies seem to move towards low energies; thus, the hump structure becomes much more visible, accompanied by a small shift of its position toward lower energies. Furthermore, the dip and hump positions shift slightly towards higher energies at low temperatures, which seems to correlate with the increase of the coherence peak energy or the SC-state gap magnitude 2∆0. The clear peak-dip-hump structure has been observed in another tun-neling experiments and demonstrated to be a characteristic feature of the ARPES spectra around (±π, 0) and (0, ±π) in the SC state, as well.4-7,11,12)On the other hand, the change in the high-energy hump structure across T c has not beenconfirmed in ARPES experiments. Therefore, it could be explained from the experimental point of view, for exam-ple, as follows: an extrinsic background, which made the clear hump structure indistinct, is superposed in the tunnel-ing spectra above T c. However, such a change in the UD tunneling spectrum across T c is reproducible in our BVB junction experiments (see Fig. 3 (c) for another UD sample) and can also be seen in the Bi2212 tunneling spectra meas-ured in the same type of junctions by Miyakawa et al.4,7) and in recent point-contact junctions by Oki et al.40)An-other explanation for the change in the high-energy hump structure across T c could also be made on the basis of a possible intrinsic origin, for example, in terms of the AF spin fluctuations; in the SC state below T c, where a coher-ent spin-singlet state is realized, the AF spin fluctuations will be suppressed, leading to the observed change in the high-energy spectrum responsible for the LPG.4. SummaryIn summary, the tunneling spectra of Bi2212 were meas-ured over a wide T range in BVB junctions fabricated using STM, and it was clearly demonstrated that, in the electronic excitations, there exist two kinds of pseudogaps, SPG and LPG; the former one (SPG) with a characteristic energy comparable to the SC-state gap and the latter one (LPG) that is 3 to 4 times larger. The SPG, which develops pro-gressively below the mean-field characteristic temperature T co for d-wave superconductors and then evolves into the SC-state gap at T c, will be some kind of precursor of su-perconductivity. On the other hand, the LPG, which re-mains open even at high temperatures above T co, seems to be related to the crossover behavior of magnetic suscepti-bility around T max (>T co), which arises from the gradual development of AF spin fluctuations. It was also found in UD Bi2212 that, in accordance with the SC transition, the characteristic feature of the spectrum at high energies changes from a broad hump structure to a clear hump and dip structure accompanied by a shift of the hump position, in addition to the rapid growth of the SC-state gap from the SPG at low energies. Further studies on such a modifica-tion in the quasiparticle excitations across T c will be needed to understand the SC transition mechanism and/or the pair-ing mechanism more profoundly.AcknowledgmentsThe authors would like to thank Prof. J. F. Zasadzinski and Dr. K. E. Gray for their helpful information about BVB junction fabrication and useful discussions. They are also grateful to Prof. F. J. Ohkawa and Dr. A. V. Balatsky for valuable discussions. This work was supported in part by Grant-in-Aids for Scientific Research on Priority Area (No. 407, "Novel Quantum Phenomena in Transition Metal Ox-ides") and on Projects (Nos. 12640327, 12740191 and 13440104) from the Ministry of Education, Culture, Sports, Science and Technology of Japan, and by the Kurata Foundation.Correspondence: M. Oda (moda@sci.hokudai.ac.jp)References1. J. M. Harris, Z. X. Shen, P. J White, D. S. Marshall,M. C. Schabel, J. N. Eckstein and I. Bozovic, Phys. Rev. B 54(1996) 15665.2. M. Oda, K. Hoya, R. Kubota, C. Manabe, N. Momono,T. Nakano and M. Ido, Physica C 281 (1997) 135.3. T. Nakano, N. Momono, M. Oda and M. Ido, J. Phys. Soc.Jpn. 67 (1998) 2622.4. N. Miyakawa, P. Guptasarma, J. F. Zasadzinski, D. G. Hinksand K. E. Gray, Phys. Rev. Lett. 80 (1998) 157.5. Ch. Renner, B. Revaz, J. Y. Genoud, K. Kadowaki and∅. Fischer, Phys. Rev. Lett. 80 (1998) 149.6. J. C. Campuzano et al., Phys. Rev. Lett. 83 (1999) 3709.7. N. Miyakawa, J. F. Zasadzinski, L. Ozyuzer, P. Guptasarma,C. Kendziora,D. G. Hinks, T. Kaneko and K.E. Gray,Physica C 341-348, (2000) 835.8. M. Ido, N. Momono and M. Oda, J. Low Temp. Phys., 117(1999) 329.9. M. Oda, R. M. Dipasupil, N. Momono and M. Ido, J. Phys.Soc. Jpn. 69 (2000) 983.10. H. Ding et al., cond-mat/0006134.11. A. G. Loeser, Z. X. Shen, D. S. Dessau, D. S. Marshall, C. H.Park, P. Fournier and A. Kapitulnik, Science 273 (1996) 325.12. H. Ding, T. Yokoya, J. C. Campuzano, T. Takahashi,M. Randeria, M. R. Norman, T. Mochiku, K. Kadowaki andJ. Giapintzakis, Nature 382 (1996) 51.13. A. Matsuda, S. Sugita and T. Watanabe, Phys. Rev. B60(1999) 1377.14. M. Sato, H. Harashina, J. Takeda, S. Yoshii, Y. Kobayashiand K. Kakurai, J. Phys. Chem. Solids 62 (2001) 7.15. V. M. Krasnov, A. Yurgens, D. Winkler, P. Delsing andT. Claeson, Phys. Rev. Lett. 84 (2000) 5860.16. M. Suzuki and T. Watanabe, Phys. Rev. Lett, 85 (2000) 4787.17. T. Sato, Y. Naitoh, T. Kamiyama, T. Takahashi, T. Yokoya,K. Yamada, Y. Endoh and K. Kadowaki, Physica C 341-348(2000) 815.18. W. A. Groen, D. M. de Leeuw and L. F. Feiner, Physica C 165(1990) 55.19. T. Ekino, Y. Sezaki and H. Fujii, Phys. Rev. B60 (1999) 6916.20. N. Nishida and H. Sakata, J. Jpn. Appl. Phys. 11 (1999) 77.21. R. E. Walstedt, R. F. Bell and D. B. Mitzi, Phys. Rev. B 44(1991) 7760.22. K. Ishida, K. Yoshida, T. Mito, Y. Tokunaga, Y. Kitaoka,K. Asayama, Y. Nakayama, J. Shimoyama and K. Kishio,Phys. Rev. B58 (1998) 5960.23. F. J. Ohkawa, J. Phys. Soc. Jpn. 56 (1987) 2267.24. H. Won and K. Maki, Phys. Rev. B 49 (1994), 1397.25. T. Tanamoto, K. Kohno and H. Fukuyama, J. Phys. Soc. Jpn.61 (1992) 1886.26. S-S Lee and S-H S. Salk, cond-mat/9907226, Phys. Rev. B 64052501 (2001).27. M. Kugler, ∅. Fischer, Ch, Renner, S. Ono and Y. Ando,Phys, Rev. Lett. 86 (2001) 4911.28. N. Momono, T. Matsuzaki, T. Nagata, M. Oda andM. Ido, J. Low Temp. Phys. 117 (1999) 353.29. V. J. Emery and S. A. Kivelson, Nature 374 (1995) 434.30. B. Janko, J. Maly and K. Levin, Phys. Rev. B56 (1997)11407, Q. J. Chen et al., Phys. Rev. Lett. 81 (1998)4708.31. Y. Yanase and K. Yamada, Phys. Soc. Jpn. 68 (1999)2999.32. I. Martin, G. Ortiz, A. V. Balatsky and A. R. Bishop,Int. J. of Mod. Phys., 14 (2000) 3567. I. Martin, G.Ortiz, A. V. Balatsky and A. R. Bishop, Europhys. Lett.56 (2001) 849.33. Z. Tesanovic, O. Vafek and M. Franz, cond-mat/0110253. M. Franz and Z. Tesanovic, Phys. Rev. Lett.87 (2001) 257003.34. D. C. Johnston, Phys. Rev. Lett. 62 (1989) 957.35. T. Nakano, M. Oda, C. Manabe, N. Momono, Y. Miuraand M. Ido, Phys. Rev. B 49 (1994) 16000.36. J. Mesot, N. Netoki, M. Bohm, A. Hiess, K. Kadowaki,Physica C 341-348 (2000) 2105, J. Mesot, M. Boehm,M. R. Norman, M. Randeria, N. Metoki, A. Kaminski,S. Rosenkranz, A. Hiess, H. M. Fretwell, J. C. Cam-puzano, K. Kadowaki, cond-mat/0102339.37. H. F. Fong, P. Bourges, Y. Sidis, L. P. Regnaut, A.Ivanov, G. D. Gu, N. Koshizuka and B. Keimer, Nature398 (1999) 1234.38. A. Lanzara et al., Nature 412 (2001) 588.39. J. F. Zasadzinski, L. Ozyuzer, N. Miyakawa,K. E. Gray, D. G. Hinks and C. Kendziora, Phys. Rev.Lett. 87 (2001) 7005.40. J. Oki, N. Tsuda and D. Shimada, Physica C 353 (2001)213.Figure CaptionsFig. 1: Schematic diagram of BVB junction (a), I-V characteristic at 5 K in a UD BVB junction (b) and the corresponding tunnel-ing spectrum (c).Fig. 2: Tunneling spectra (d I/d V-V curves) in the BVB junctions of OD p~0.21 and UD p~0.14 Bi2212 samples. The spectrum ateach temperature is normalized with the value at –150 mV.The zero level in the vertical axis is only for the low-est-temperature spectrum, and the other spectra are shifted up-wards for clarity.Fig. 3: 3D illustrations of the tunneling spectra for p~0.21, 0.14 and 0.12, which were generated by using 3D imaging software.The T c value, indicated in this figure, was determined fromdc-SQUID diamagnetic susceptibility measurements.Fig. 4: T dependence of normalized σ(0) (zero-bias conductance).The σ(0) data for p~0.12, 0.14 and 0.21, represented by closedcircles, are plotted from the tunneling spectra in Figs. 3 (a), (b)and (c), respectively. For p~0.14, the σ(0) data measured inanother BVB junction above ~100 K are also shown by opencircles, together with those in Fig. 3 (b), to demonstrate that theT dependences of σ(0) in both junctions are qualitatively con-sistent with each other. The arrows represent the T c valuesdetermined from dc-SQUID diamagnetic susceptibility meas-urements.Fig. 5: Dependences on 2∆0, T*, T co and T c on p in Bi2212.Temperature scale is taken so that the 2∆0 data give the T co val-ues. The inset shows the p-dependence of 2∆0/k B T*, whichwas obtained from the tuneling T* and low-tempertature(T<<T c) tunneling gap 2∆0.Fig. 6: Temperature dependence of the dip depth defined as in the inset. The data were obtained from two UD (p~0.14) BVBjunctions and normalized with the lowest-temperature value.。