The Design of a Performance Steering System for Component-based Grid Applications
汽车转向系统外文原文及翻译

本文摘于《Race Car Vehicle Dynamics》作者:William F. Miliken and Douglas L. MilikenSteering systemsIntroductionThis chapter begins with a discussion of steering geometry—casterangle ,trail ,kingpin inclination ,and scrub radius .The next section discuss Ackermann geometry followed by steering racks and gears .Ride steer (bump steer ) and roll steer are closely related to each other ;without compliance they would be thesame .Finally ,wheel alignment is discussed .this chapter is tied to chapter 17 on suspension geometry –when designing a new chassis ,steering and suspension geometry considerations are high priorities .19.1 steering geometryThe kingpin in a solid front axle is the steering pivot .In modern independent suspensions , introduced by Maurice olley at Cadillac in 1932,the kingpin is replaced by two (or more ) ball joints that define the steering axis .This axis is not vertical or centered on the tire contact patch for a number of reason .see figure 19.1 to clarify how kingpin location is measured .In front view ,the angle is called kingpin inclination and the offset of the steering axis from the center of the tire print measured along the ground is called scrub (or scrub radius ). The distance from the kingpin axis to the wheel center plane , measured horizontally at axle height ,is the spindle length .In side view the kingpin angle is called caster angle ; if the kingpin axis does not pass through the wheel center then side view kingpin offset is present ,as in most motorcycle front ends .The distance measured on the ground from the steering axis to the center of the tire print is the trail (called caster offset in ref .1 )Kingpin front view geometryAs mentioned in chapter 17, kingpin inclination ,spindle length ,and scrub are usually a compromise between packaging and performance requirements .Some factors to consider include :1.With a positive spindle length (virtually every car is positive as shown in figure 19.1) the car will be raised up as the wheels are steered away from center .The more the kingpin inclination is tilted from vertical the more the car will be raised when the front wheels are steered .This effect always raises the car , regardless of which direction the wheel is steered ,unless the kingpin inclination is truevertical .the effect is symmetric side to side only if there is no caster angle .See the following section on caster angle .For a given kingpin inclination ,a longer positive spindle length will increase the amount of lift with steer .2.The effect of kingpin inclination and spindle length in raising the front end ,by itself ,is to aid centering of the steering at low speed .At high speed any trail will probably swamp out the effect that raise ad fall have on centering .3. Kingpin inclination affects the steer –camber characteristic .when a wheel is steered ,it will lean out at the top ,toward positive camber ,if the kingpin is inclined in the normal direction (toward the center of the car at the upper end ). Positive camber results for both left– and right-hand steer .the amount of this effect is small ,but significant if the track includes tight turns.4. When a wheel is rolling over a bumpy road ,the rolling radius is constantly changing ,resulting in changes of wheel rotation speed . This gives rise to longitudinal forces at the wheel center .The reaction of these forces will introduce kickback into the steering in proportion to the spindle length .If the spindle length is zero then there will be no kick from this source .Design changes made in the last model of the GM “P ”car (fiero ) shortened the spindle length and this resulted in less wheel kickback on rough roads when compared to early model “P ”cars.5. The scrub radius shown in figure 19.1 is negative ,as used on front-wheel–drive cars (see below ) . driving or braking forces (at the ground ) introduce steer torques proportional to the scrub radius . If the driving or braking force is different on left and right wheels then there will be a net steering torque felt by the driver (assuming that the steering gear has good enough rev erse efficiency ).The only time that this is not true is with zero scrub (centerpoint steering ) because there is no moment arm for the drive (or brake ) force to generate torque about the kingpin .With very wide tires the tire forces often are not centered in the wheel center plane due to slight changes in camber ,road surface irregularities ,tire nonuniformity (conicity ),or other asymmetric effects .These asymmetries can cause steering kickback regardless of the front view geometry .Packaging requirements often conflict with centerpoint steering and many race cars operate more or less okay on smooth tracks with large amounts of scrub .6. For front drive ,a negative scrub radius has two strong stabilizingeffects :first ,fixed steering wheel –if one drive wheel loses traction ,the opposingwheel will toe –out an amount determined by the steer compliance in the system .This will tend to steer the car in a straight line ,even though the tractive force is not equal side-to –side and the unequal tractive force is applying a yaw moment to the vehicle .Second ,with good reverse efficiency the driver’s hands never truly fix the steering wheel . In this case the steering wheel may be turned by the effect of uneven longitudinal tractive forces ,increasing the stabilizing effect of the negative scrub radius .Under braking the same is true .Negative scrub radius tends to keep the car traveling straight even when the braking force is not equal on the left and right side front tiresome (due to differences in the roadway or the brakes).Caster angle and trailWith mechanical trail ,shown in figure 19.1,the tire print follows behind the steering axis in side view .Perhaps the simplest example is on an office chair caster–with any distance of travel ,the wheel aligns itself behind the point .More trail means that the tire side force has a large moment arm to act on the kingpin axis .This produces more self-centering effect and is the primary source of self-centering moment about the kingpin axis at speed .Some considerations for choosing the caster angle and trail are :1.More trail will give higher steering force .with all cars ,less trail will lower the steering force .In some cases ,manual steering can be used on heavy sedans (instead of power steering ) if the trail is reduced to almost zero .2.Caster angle ,like kingpin inclination ,cause the wheel to rise and fall with steer .unlike kingpin inclination ,the effect is opposite from side to side .With symmetric geometry (including equal positive caster on left and right wheels ) ,the effect of left steer is to roll the car to the right ,causing a diagonal weight shift .In this case ,more load will be carried on the LF –RR diagonal ,an oversteer effect in aleft-hand turn .The diagonal weight shift will be larger if stiffer springing is used because this is a geometric effect .The distance each wheel rises (or falls ) is constant but the weight jacking and chassis roll angle are functions of the front and rear roll stiffness. This diagonal load change can be measured with the car on scales and alignment ( weaver ) plates .Keep in mind that the front wheels are not steered very much in actual racing , except on the very tightest hairpin turns . For example , on a 100-ft .radius (a 40-50 mph turn ), a 10-ft. wheelbase neutral steer car needs only about 0.1rad .(5.7)of steer at the front wheels (with a 16:1steering ratio this is about 90degree at the steering wheel ).For cars that turn in one direction only , caster stagger (differences in left and right caster ) is used to cause the car to pull to one side due to the car seeking the lowest ride height . caster stagger will also affect the diagonal weight jacking effect mentioned above .If the caster is opposite (positive on one side and negative the same number of degrees on the other side ) then the front of the car will only rise and fall with steer ,no diagonal weight jacking will occur .3. Caster angle affects steer-camber but ,unlike kingpin inclination ,the effect is favorable . With positive caster angle the outside wheel will camber in a negative direction (top of the wheel toward the center of the car ) while the inside wheel cambers in a positive direction , again learning into the turn .In skid recovery , “opposite lock ” (steer out of the turn ) is used and in this case the steer–camber resulting from caster angle is in the “wrong ” direction for increased front tire grip . conveniently ,this condition results from very low lateral force at the rear so large amounts of front grip are not needed .4. As discussed in chapter 2, tires have pneumatic trail which effectively adds to (and at high slip Angles subtracts from ) the mechanical trail . This tire effect is nonlinear with lateral force and affects steering torque and driver feel .In particular , the fact that pneumatic trail approaches zero as the tire reaches the limit will result in lowering the self-centering torque and can be s signal to the driver that the tire is near breakaway .The pneumatic trail “breakaway signal” will be swamped out by mechanical trail if the mechanical trail is large compared to the pneumatic trail .5.Sometimes the trail is measured in a direction perpendicular to the steering axis (rather than horizontal as shown in figure 19.1) because this more accurately describes the lever (moment ) arm that connects the tire lateral forces to the kingpin . Tie rod locationNote that in figure 19.1 a shaded area is shown for the steering tie rod location . Camber compliance under lateral force is unavoidable and if the tie rod is located as noted ,the effect on the steering will be in the understeer ( steer out of the turn ) direction becomes much more complex than can be covered here .19.2 Ackerman steering geometryAs the front wheels of a vehicle are steered away from the straight-ahead position ,the design of the steering linkage will determine if the wheels stay parallel or if one wheel steers more than the other .This difference in steer Angles on the left and right wheels should not be confused with toe-in or toe-out which are adjustments and add to ( or subtract from ) Ackerman geometric effects .For low lateral acceleration usage (street cars) it is common to use Ackerman geometry . as seen on the left of figure 19.2, this geometry ensures that all the wheels roll freely with no slip Angles because the wheels are steered to track a common turn center . Note that at low speed all wheels are on a significantly different radius , the inside front wheel must steer more than the outer front wheel . A reasonable approximation to this geometry may be as shown in figure 19.3.According to ref .99, Rudolf Ackerman patented the double pivot steering system in 1817 and in 1878, Charles Jeantaud added the concept mentioned above to eliminate wheel scrubbing when cornering . Another reason for Ackermann geometry ,mentioned by Maurice olley , was to keep carriage wheels from upsetting smooth gravel driveways .High lateral accelerations change the picture considerably . Now the tires alloperate at significant slip Angles and the loads on the inside track are less than on the outside track . Looking back to the tire performance curves ,it is seen that less slip angle is required at lighter loads to reach the peak of the cornering force to a higher slip angle than required for maximum side force . Dragging the inside tire along at high slip Angles ( above for peak lateral force ) raise the tire temperature and slows the car down due to slip angle ( induced ) drag .For racing , it is common to use parallel steering or even reverse Ackermann as shown on the center and right side of figure 19.2.It is possible to calculate the correct amount of reverse Ackermann if the tire properties and loads are known . In most cases the resulting geometry is found to be too extreme because the car must also be driven (or pushed ) at low speeds , for example in the pits .Another point to remember is that most turns in racing have a fairly large radius and the Ackermann effect is very small . In fact , unless the steering system and suspension are very stiff ,compliance (deflection ) under cornering loads may steer the wheels more than any Ackermann (or reverse Ackermann ) built into the geometry .The simplest construction that generates Ackermannn geometry is shown in figure 19.3 fo r “rear steer ” . Here ,the rack (cross link or relay rod in steering box systems ) is located behind the front axle and lines staring at the kingpin axis , extended through the outer tie rod ends , intersect in the center of the rear axle . The angularity of the steering knuckle will cause the inner wheel to steer more than the outer (toe-out on turning ) and a good approximation of “perfect Ackermann ” will be achieved .The second way to design-in differences between inner and outer steer Angles is by moving the rack (or cross link ) forward or backward so that it is no longer on a line directly connecting the two outer tie rod ball joints .This is shown in figure 19.4. with “rear steer ” , as shown in the figure ,moving the rack forward will tend mo re toward parallel steer (and eventually reverse Ackermann ), and moving it toward the rear of the car will increase the toe-out on turning .A third way to generate toe with steering is simply to make the steering arms different lengths . A shorter steering arm (as measured from the kingpin axis to the outer tie rod end ) will be steered through a larger angle than one with a longer knuckle. Of course this effect is asymmetric and applies only to cars turning in one direction—oval track cars .RecommendationWith the conflicting requirements mentioned above , the authors feel that parallel steer or a bit of reverse Ackermann is a reasonable compromise . With parallel steer , the car will be somewhat difficult to push through the pits because the front wheels will be fighting each other . at racing speeds , on large-radius turns , the front wheels are steered very little , thus any ackermann effects will not have a large effect on the individual wheel slip angles , relative to a reference steer angle , measured at the centerline of the car .文献翻译摘自《Race Car Vehicle Dynamics》第19章转向系统序言:本章以转向几何参数的讨论为开始,包括主销后倾角,后倾拖距,主销内倾角,主销偏置量。
美国高速公路安全新车评测项目之侧翻控制(英文)

NHTSA’S NCAP ROLLOVER RESISTANCE RATING SYSTEMPatrick L. BoydNational Highway Traffic Safety Administration United StatesPaper Number 05-0450ABSTRACTStarting in the 2004 model year, the National Highway Traffic Safety Administration (NHTSA) improved the rollover resistance ratings in its New Car Assessment Program (NCAP) consumer information by adding a dynamic maneuver test. NHTSA had provided rollover resistance ratings in the 2001 – 2003 model years based solely on the Static Stability Factor (SSF) measurement of vehicles. The ratings express the risk of a vehicle rolling over in the event of a single vehicle crash, the type of crash in which most rollovers occur. The SSF, which is determined by a vehicle’s center of gravity height and track width, had proved to be a powerful predictor of rollover risk based on a linear regression study of rollover rates of 100 vehicle models in 224,000 single vehicle crashes (R2 = 0.88). The TREAD Act required NHTSA to change its rollover resistance ratings to use a dynamic maneuver test, and the 2004 and later NCAP rollover resistance ratings use both SSF and a dynamic maneuver. This paper describes the development of the risk prediction model used for present rating system. Twenty-five vehicles were tested using two highly objective automated steering maneuvers (J-turn and Fishhook) at two levels of passenger loading. A logistic regression risk model was developed based on the rollover outcomes of 86,000 single-vehicle crashes involving the make/models that were tested. The vehicles were characterized by their SSF measurements and binary variables indicating whether or not they had tipped up during the maneuver tests. It was found that the Fishhook test in the heavy (5 passenger equivalent) load was the most useful maneuver test for predicting rollover risk. The relative predictive powers of the SSF measurement and the Fishhook test were established by a logistic regression model operating on the rollover outcomes of real-world crash data. This model was used to predict the rollover rates of vehicles in the 2004 and 2005 NCAP program based on their SSF measurements and Fishhook maneuver test performance. The information in this paper first appeared in NHTSA’s Federal Register notice [1] that established the NCAP rollover resistance rating system for model year 2004. INTRODUCTIONPrior NCAP Program and the TREAD ActNHTSA’s NCAP program has been publishing comparative consumer information on frontal crashworthiness of new vehicles since 1979, on side crashworthiness since 1997, and on rollover resistance since January 2001.The 2001-2003 NCAP rollover resistance ratings were based on the Static Stability Factor (SSF) of a vehicle, which is the ratio of one half its track width to its center of gravity (C.G.) height. After an evaluation of some driving maneuver tests in 1997 and 1998, NHTSA chose to use SSF instead of any driving maneuvers to characterize rollover resistance. NHTSA chose SSF as the basis of NCAP ratings because it represents the first order factors that determine vehicle rollover resistance in the vast majority of rollovers which are tripped by impacts with curbs, soft soil, pot holes, guard rails, etc. or by wheel rims digging into the pavement. In contrast, untripped rollovers are those in which tire/road interface friction is the only external force acting on a vehicle that rolls over. Driving maneuver tests directly represent on-road untripped rollover crashes, but such crashes represent less than five percent of rollover crashes [2].At the time, NHTSA believed it was necessary to choose between SSF and driving maneuver tests as the basis for rollover resistance ratings. SSF was chosen because it had a number of advantages: it is highly correlated with actual crash statistics; it can be measured accurately and inexpensively and explained to consumers; and changes in vehicle design to improve SSF are unlikely to degrade other safety attributes. NHTSA also considered the fact that an improvement in SSF represents an increase in rollover resistance in both tripped and untripped circumstances while maneuver test performance can be improved by reduced tire traction and certain implementations of electronic stability control that it believes are much less likely than SSF improvements to increase resistance to tripped rollovers.Congress directed the agency to enhance the NCAP rollover resistance rating program. Section 12 of the “Transportation Recall, Enhancement, Accountability and Documentation (TREAD) Act of November 2000" directs the Secretary to “develop a dynamic test on rollovers by motor vehicles for a consumer information program; and carry out a program conducting such tests. As the Secretary develops a [rollover] test, the Secretary shall conduct a rulemaking to determine how best to disseminate test results to the public.” The rulemaking was to be carried out by November 1, 2002.Research and Public Comment on Dynamic Rollover TestsOn July 3, 2001, NHTSA published a Request for Comments notice (66 FR 35179) regarding its research plans to assess a number of possible dynamic rollover tests. The notice discussed the possible advantages and disadvantages of various approaches that had been suggested by manufacturers, consumer groups, and NHTSA’s prior research. The driving maneuver tests to be evaluated fit into two broad categories: closed-loop maneuvers in which all test vehicles attempt to follow the same path, and open-loop maneuvers in which all test vehicles are given equivalent steering inputs. The principal theme of the comments was a sharp division of opinion about whether the dynamic rollover test should be a closed loop maneuver test like the ISO 3888 double lane change that emphasizes the handling properties of vehicles or whether it should be an open loop maneuver like a J-Turn or Fishhook that are limit maneuvers in which vulnerable vehicles would actually tip up. Ford recommended a different type of closed loop lane change maneuver in which a path-following robot or a mathematical correction method would be used to evaluate all vehicles on the same set of paths at the same lateral acceleration. It used a measurement of partial wheel unloading without tip-up at 0.7g lateral acceleration as a performance criterion in contrast to the other closed loop maneuver tests that used maximum speed through the maneuver as the performance criterion. Another unique comment was a recommendation from Suzuki to use a sled test developed by Exponent Inc. to simulate tripped rollovers.The subsequent test program [3] (using four SUVs in various load conditions and with and without electronic stability control enabled on two of the SUVs) showed that open-loop maneuver tests using an automated steering controller could be performed with better repeatability of results than the other maneuver tests. The J-Turn maneuver and the Fishhook maneuver (with steering reversal at maximum vehicle roll angle) were found to be the most objective tests of the susceptibility of vehicles to maneuver-induced on-road rollover. Except for the Ford test, the closed loop tests were found not to measure rollover resistance. Instead, the evaluation criterion of maximum maneuver entrance speed measured just prior to entering a double lane change assessed vehicle agility. None of the test vehicles tipped up during runs in which they maintained the prescribed path even when loaded with roof ballast to experimentally reduce their rollover resistance. The speed scores of the test vehicles in the closed loop maneuvers were found to be unrelated to their resistance to tip-up in the open-loop maneuvers that actually caused tip-up. The test vehicle that was clearly the poorest performer in the maneuvers that caused tip-ups achieved the best score (highest speed) in the ISO 3888 and CU short course double lane change, and one vehicle improved its score in the ISO 3888 test when roof ballast was added to reduce its rollover resistance.Due to the non-limit test conditions and the averaging necessary for stable wheel force measurements, the wheel unloading measured in the Ford test appeared to be more quasi-static (as in driving in a circle at a steady speed or placing the vehicle on a centrifuge) than dynamic. Sled tests were not evaluated because NHTSA believed that SSF already provided a good indicator of resistance to tripped rollover. National Academy of Sciences StudyDuring the time NHTSA was evaluating dynamic maneuver tests in response the TREAD Act, the National Academy of Sciences (NAS) was conducting a study of the SSF-based rollover resistance ratings and was directed to make recommendations regarding driving maneuver tests. NHTSA expected the NAS recommendations to have a strong influence on TREAD-mandated changes to NCAP rollover resistance ratings.When NHTSA proposed the prior (SSF only) rollover resistance ratings in June 2000, vehicle manufacturers generally opposed it because they believed that SSF as a measure of rollover resistance is too simple since it does not include the effects of suspension deflections, tire traction and electronic stability control (ESC). In addition, the vehicle manufacturers argued that the influence of vehicle factors on rollover risk is too slight to warrant consumer information ratings for rollover resistance. In the conference report of the FY2001 DOT Appropriations Act, Congress permitted NHTSA tomove forward with its rollover rating program, but directed the agency to fund a National Academy of Sciences (NAS) study on vehicle rollover ratings. The study topics were “whether the static stability factor is a scientifically valid measurement that presents practical, useful information to the public including a comparison of the static stability factor test versus a test with rollover metrics based on dynamic driving conditions that may induce rollover events.” The National Academy’s report was completed and made available at the end of February 2002 [4].The NAS study found that SSF is a scientifically valid measure of rollover resistance for which the underlying physics and real-world crash data are consistent with the conclusion that an increase in SSF reduces the likelihood of rollover. It also found that dynamic tests should complement static measures, such as SSF, rather than replace them in consumer information on rollover resistance. The dynamic tests the NAS recommended would be driving maneuvers used to assess “transient vehicle behavior leading to rollover.”The NAS study also made recommendations concerning the statistical analysis of rollover risk and the representation of ratings. It recommended that NHTSA use logistic regression rather than linear regression for analysis of the relationship between rollover risk and SSF, and it recommended that NHTSA consider a higher-resolution representation of the relationship between rollover risk and SSF than is provided by a five-star rating system. NHTSA published a Federal Register notice on October 7, 2002 (67 FR 62528) that proposed to modify the NCAP rollover resistance ratings to satisfy the requirements of the TREAD Act and to align it with the recommendation of the NAS report. NHTSA chose the J-Turn and Fishhook maneuver (with roll rate feedback) as the dynamic maneuver tests because they were the type of limit maneuver tests that could directly lead to rollover as recommended by the NAS. NHTSA also proposed to use a logistic regression analysis to determine the relationship between vehicle properties and rollover risk, as recommended by the NAS.DYNAMIC MANEUVER TESTS OF 25 VEHICLESThe original NCAP rollover resistance ratings predicted the rate of rollovers per single vehicle crash based on the SSF of vehicles. Stars were used to express rollover risk in rate increments of 10% (i.e., 2 stars for a predicted rollover rate between 30 and 40%, 3 stars for a predicted rollover rate between 20 and 30%, etc.). The relationship between rollover rate and SSF was determined using a linear regression between the logarithm of SSF and the actual rollover rates of 100 vehicle make/models [5]. The rollover rates were determined from 224,000 state crash reports and were corrected for differences between vehicles in demographic and road condition variables reported by the states.The idea for improving the prediction of rollover rate (the risk model) using dynamic maneuver tests was to describe the vehicle by its SSF plus a number of variables resulting from the vehicle’s behavior in the dynamic maneuvers. In that way, the risk model would consider more than just the geometric properties of the vehicle. Four binary variables were anticipated. They would describe whether the vehicle tipped up or did not in the J-turn and in the Fishhook maneuver, each performed with the vehicle in two passenger load configurations. The risk model for predicting rollover rate on the basis of SSF plus dynamic test results would be determined using logistic regression between the rollover outcomes of state crash reports of single vehicle crashes of a number of vehicles and the new set of vehicle attributes (SSF plus dynamic test variables). The expression of rollover risk by stars would continue with the same relationship between the number of stars and the predicted rollover rate.The linear regression, SSF only, risk model used crash data on 100 vehicles, but it was impractical to perform maneuver tests on that many vehicles to develop the present risk model. This section presents an overview of the test maneuvers and the results for the subset of 25 vehicles selected for developing the logistic regression risk model. A more extensive account of the test program is contained in the Phase VI and VII rollover research report [6]. The NHTSA J-Turn and Fishhook (with roll rate feedback) maneuver tests were performed for 25 vehicles representing four vehicle types including passenger cars, vans, pickup trucks and SUVs. NHTSA chose mainly high production vehicles that spanned a wide range of SSF values, using vehicles NHTSA already owned where possible. Except for four 2001 model year vehicles NHTSA purchased new, the vehicle suspensions were rebuilt with new springs and shock absorbers, and other parts as required for all the other vehicles included in the test program.J-Turn ManeuverThe NHTSA J-Turn maneuver represents anavoidance maneuver in which a vehicle is steered away from an obstacle using a single input. The maneuver is similar to the J-Turn used during NHTSA’s 1997-98 rollover research program and is a common maneuver in test programs conducted by vehicle manufacturers and others. Often the J-Turn is conducted with a fixed steering input (handwheel angle) for all test vehicles. In its 1997-98 testing, NHTSA used a fixed handwheel angle of 330 degrees. During the development of the present tests, NHTSA developed an objective method of specifying equivalent handwheel angles for J-Turn tests of various vehicles, taking into account their differences in steering ratio, wheelbase and linear range understeer properties [3]. Under this method, one first measures the handwheel angle that would produce a steady-state lateral acceleration of 0.3 g at 50 mph on a level paved surface for a particular vehicle. In brief, the 0.3 g value was chosen because the steering angle variability associated with this lateral acceleration is quite low and there is no possibility that stability control intervention could confound the test results. Since the magnitude of the handwheel position at 0.3 g is small, it must be multiplied by a scalar to have a high maneuver severity. In the case of the J-Turn, the handwheel angle at 0.3 g was multiplied by eight. When this scalar is multiplied by handwheel angles commonly observed at 0.3 g, the result is approximately 330 degrees. Figure 1 illustrates the J-Turn maneuver in terms of the automated steering inputs commanded by the programmable steering machine. The rate of the handwheel turning is 1000 degrees per second. To begin the maneuver, the vehicle was driven in astraight line at a speed slightly greater than the desired entrance speed. The driver released the throttle, coasted to the target speed, and then triggered the commanded handwheel input. The nominal maneuver entrance speeds used in the J-Turn maneuver ranged from 35 to 60 mph, increased in 5 mph increments until a termination condition was achieved. Termination conditions were simultaneous two inch or greater lift of a vehicle’s inside tires (two-wheel lift) or completion of a test performed at the maximum maneuver entrance speed without two-wheel lift. If two-wheel lift was observed, a downward iteration of vehicle speed was used in 1 mph increments until such lift was no longer detected. Once the lowest speed for which two-wheel lift could be detected was isolated, two additional tests were performed at that speed to monitor two-wheel lift repeatability.Fishhook ManeuverThe Fishhook maneuver uses steering inputs that approximate the steering a driver acting in panic might use in an effort to regain lane position after dropping two wheels off the roadway onto the shoulder. NHTSA has often described it as a road edge recovery maneuver. As pointed out by some commenters, it is performed on a smooth pavement rather than at a road edge drop-off, but its rapid steering input followed by an over-correction is representative of a general loss of control situation. The original version of this test was developed by Toyota, and variations of it were suggested by Nissan and Honda. NHTSA has experimented with several versions since 1997, and the present test includes roll rate feedback in order to time the counter-steer to coincide with the maximum roll angle of each vehicle in response to the first steer.Figure 2 describes the Fishhook maneuver in terms of the automated steering inputs commanded by the programmable steering machine and illustrates the roll rate feedback. The initial steering magnitude and countersteer magnitudes are symmetric, and are calculated by multiplying the handwheel angle that would produce a steady state lateral acceleration of 0.3 g at 50 mph on level pavement by 6.5. When this scalar is multiplied by handwheel angles commonly observed at 0.3 g, the result is approximately 270 degrees. This is equivalent to the 270 degree handwheel angle used in earlier forms of the maneuver but, as in the case of the J-Turn, the procedure above is an objective way of compensating for differences in steering gear ratio, wheelbase and understeer properties between vehicles. The fishhook maneuver dwell times (the time between completion of the initial steering ramp and the initiation of the countersteer) are defined by the roll motion of the vehicle being evaluated, and can vary on a test-to-test basis. This is made possible by having the steeringFigure 1. NHTSA J-turn maneuver description.machine monitor roll rate (roll velocity). If an initial steer is to the left, the steering reversal following completion of the first handwheel ramp occurs when the roll rate of the vehicle first equals or goes below 1.5 degrees per second. If an initial steer is to the right, the steering reversal following completion of the first handwheel ramp occurs when the roll rate of the vehicle first equals or exceeds -1.5 degrees per second. The handwheel rates of the initial steer and countersteer ramps are 720 degrees per second.To begin the maneuver, the vehicle was driven in astraight line at a speed slightly greater than the desired entrance speed. The driver released the throttle, coasted to the target speed, and then triggered the commanded handwheel input described in Figure 2. The nominal maneuver entrance speeds used in the fishhook maneuver ranged from 35 to 50 mph, increased in 5 mph increments until a termination condition was achieved. Termination conditions included simultaneous two inch or greater lift of a vehicle’s inside tires (two-wheel lift) or completion of a test performed at the maximum maneuver entrance speed without two-wheel lift. If two-wheel lift was observed, a downward iteration of vehicle speed was used in 1 mph increments until such lift was no longer detected. Once the lowest speed for which two-wheel lift could be detected was isolated, two additional tests were performed at that speed to check two-wheel lift repeatability.NHTSA observed that during the Fishhook tests, excessive steering caused some vehicles to reach their maximum roll angle response to the initial steering input before it had been fully completed (this is essentially equivalent to a “negative” T1 in Figure 2). Since dwell time duration can have a significant effect on how the Fishhook maneuver’s ability to produce two-wheel lift, excessive steering may stifle the most severe timing of the counter steer for some vehicles. In an attempt to better insure high maneuver severity, a number of vehicles that did not produce two-wheel lift with steering inputs calculated with the 6.5 multiplier were also tested with lesser steering angles by reducing the multiplier to 5.5. This change increased the dwell times observed during the respective maneuvers. Some vehicles tipped up in Fishhook maneuvers conducted at the lower steering angle (5.5 multiplier) but not at the higher steering angle (6.5 multiplier). NHTSA adopted the practice of performing Fishhook maneuvers at both steering angles for NCAP. Loading ConditionsThe vehicles were tested in each maneuver in two load conditions in order to create four levels of stringency in the suite of maneuver tests. The light load was the test driver plus instrumentation in the front passenger seat, which represented two occupants. A heavier load was used to create a higher level of stringency for each test. In our NPRM, NHTSA announced that the heavy load would include 175 lb anthropomorphic forms (water dummies) in all rear seat positions. During the test of the 25 vehicles, it became obvious that heavy load tests were being run at very unequal load conditions especially between vans and other vehicles (two water dummies in some vehicles but six water dummies in others). While very heavy passenger loads can certainly reduce rollover resistance and potentially cause special problems, crashes at those loads are too few to greatly influence the overall rollover rate of vehicles. Over 94% of van rollovers in our 293,000 crash database occurred with five or fewer occupants, and over 99% of rollovers of other vehicles occurred with five or fewer occupants. The average passenger load of vehicles in our crash database was less than two: 1.81 for vans; 1.54 for SUVs; 1.48 for cars; and 1.35 for pickup trucks. In order to use the maneuver tests to predict real-world rollover rates, it seemed inappropriate to test theFigure 2. NHTSA Fishhook maneuver description.vehicles under widely differing loads that did not correspond to the real-world crash statistics. Therefore, the tests used to develop a statistical model of rollover risk were changed to a uniform heavy load condition of three water dummies (representing a 5-occupant loading) for all vehicles capable of carrying at least five occupants. Some vehicles were loaded with only two water dummies because they were designed for four occupants. For pickup trucks, water dummies were loaded in the bed at approximately the same height as a passenger in the front seat.Test ResultsThe test results in Table 1 (presented on the next page) reflect the performance as described for a heavy load condition representing five occupants except for the Ford Explorer 2DR, the Chevrolet Tracker and Metro that were designed for only four occupants, and the Honda CRV, Honda Civic and Chevrolet Cavalier that could not be loaded to the 5-occupant level without exceeding a gross axle weight rating because of the additional weight of the outriggers.Each test vehicle in Table 1 represented a generation of vehicles whose model year range is given. Twenty-four of the vehicles were taken from 100 vehicle groups whose 1994-98 crash statistics in six states were the basis of the present SSF based rollover resistance ratings. The nominal SSFs used to describe the vehicle groups in the prior statistical studies are given. While there were some variations between the SSFs of the individual test vehicles and the nominal vehicle group SSF values, the nominal SSFs were retained for the present statistical analyses because they represent vehicles produced over a wide range of years in many cases and provide a simple comparison between the risk model presented in this notice and that discussed in the previous notices. The X’s under the various test maneuver names indicate which vehicles tipped up during the tests. Eleven of the twenty-five vehicles tipped up in the Fishhook maneuver conducted in the heavy condition. The heavy condition represented a five-occupant load for all vehicles except the six mentioned above that were limited to a four-occupant load by the vehicle seating positions and GVWR. All eleven were among the sixteen test vehicles with SSFs less than 1.20. None of the vehicles with higher SSFs tipped up in any test maneuver. The Fishhook test under the heavy load clearly had the greatest potential to cause tip-up. The groups of vehicles that tipped up in other tests were subsets of the larger group of eleven that tipped up in the Fishhook Heavy test. There were seven vehicles in the group that tipped up in the J-Turn Heavy test, six of which also tipped up in the Fishhook Light test. The J-Turn Light test had the least potential to tip up vehicles. Only three vehicles tipped up, all of which had tipped up in every other test.ROLLOVER RISK MODELIn its study of NHTSA’s rating system for rollover resistance [4], the National Academy of Sciences (NAS) recommended that NHTSA use logistic regression rather than linear regression for analysis of the relationship between rollover risk and SSF. Logistic regression has the advantage that it operates on every crash data point directly rather than requiring that the crash data be aggregated by vehicle and state into a smaller number of data points. For example, NHTSA now has state data reports of about 293,000 single-vehicle crashes of the hundred vehicle make/models (together with their corporate cousins) whose single-vehicle crashes NHTSA have been tracking in six states. The logistic regression analysis of this data would have a sample size of 293,000, producing a narrow confidence interval on the repeatability of the relationship between SSF and rollover rate. In contrast, the linear regression analysis operates on the rollover rate of the hundred vehicle make/models in each of the six states. It produces a maximum sample size of only 600 (100 vehicles times six states) minus the number of samples for which fewer than 25 crashes were available for determining the rollover rate (a data quality control practice). Confidence limits computed for a data sample size of 600 will be much greater than those based on a sample size of 293,000. On average, each sample in the linear regression analysis was computed from over 400 crash report samples. However, ordinary techniques to compute the confidence intervals of linear regression results do not take into account the actual sample size represented by aggregated data. The statistical model created to combine SSF and dynamic test information in the prediction of rollover risk was computed by means of logistic regression as recommended by the NAS. Logistic regression is well suited to the correlation with crash data of vehicle properties that include both continuous variables like SSF and binary variables like tip-up or no tip-up in maneuver tests.NHTSA had previously considered logistic regression during the development of the SSF based rating system [4], but found that it consistently under-predicted the actual rollover rate at the low end of the。
四轮独立驱动独立转向电动汽车悬架和转向机构设计本科生毕业论文
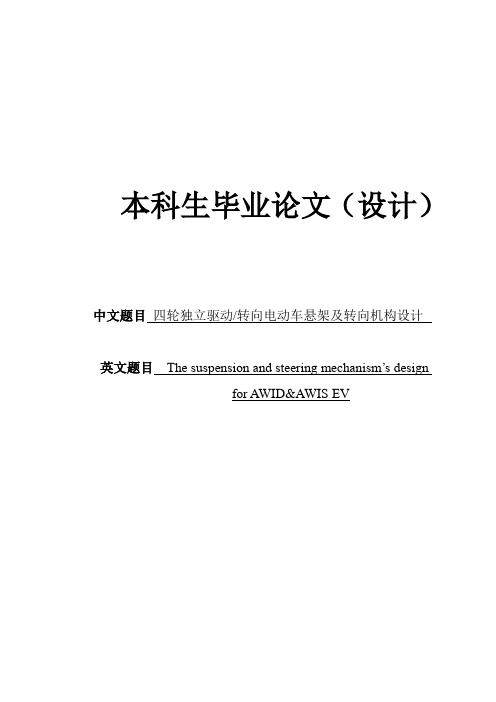
3.更具我们的结构特点,选用了双横臂弹簧减震悬架机构。简易的选择了控制臂的空间位置形式,并根据经验设计了控制臂的尺寸,校验了连接点的强度。计算并选择了合适的液压阻尼器和螺旋弹簧。
关键字:四轮独立四轮转向轮毂电机驱动轮边线控转向双横臂悬架螺栓弹簧液压减震
1.1
电动汽车四轮独立驱动系统是利用四个独立控制的电动机分别驱动汽车的四个车轮,车轮之间没有机械传动环节。典型四轮驱动布置型式,其电动机与车轮之间可以是轴式联接也可以将电动机嵌入车轮成为轮式电机,车轮一般带有轮边减速器。这种驱动系统与传统汽车驱动系统相比有以下特点:
1)传动系统得到减化,整车质量大大减轻。由电动机直接驱动车轮甚至两者集成为一体。这样省掉了离合器、变速器及传动轴等传动环节,传动效率得到提高,也更便于实现机电一体化。另外,由于动力传动的中间环节减少,传动系的振动及噪声得到改善。甚至在采用纯电力驱动时,可实现无声行驶。但是,在实际实际交通环境中无声行驶,带来的很多问题,在汽车系统没有实现完全的智能化前,带来的往往祸大于福。
1.2
在一般汽车,以操纵方向盘使前轮的轮胎转向发挥转弯机能,但四轮转向是后轮的轮胎也可转向之系统。四轮转向的目的:在低速行驶时作逆相转向(前轮与旋转方向为逆向)使旋转时小转弯性能良好,中高速时为同相转向(前轮与旋转方向为同方向),以提高在高速时之车道变换或旋转时操纵稳定性。
1)四轮转向降低低速转向半径。
如图1-1a所示,汽车在低速旋转时,车辆行进方向与轮胎方向大概可视为一致,在各轮大部份不会产生旋转向心力(cornering force )。四轮行进方向的垂直线会交于一点,车辆就以该点为中心(旋转中心)旋转。参考下图低速旋转时之行车轨迹,单轴转向车(通常前轮转向)时,因为后轮不转向,旋转中心差不多在后轴的延长线上。
铁道车辆专业英语课文翻译

枕木sleeper轨枕crossing平交道口multiple unti动车组high-speed railways高速铁路maglev磁力悬浮火车centrifugal force 离心力emergency brake handle 紧急制动手柄metro地铁light rail轻轨铁路commuter train通勤车tanker罐车operation 运转操作infrastructure下部构造platform站台EMU电力牵引动车组DMU内燃牵引动车组cushioning减震缓冲electricity-air control 电空控制antiskid防滑装置bolster枕rotational resistance回转阻hunting蛇行narrow-gauge窄轨bolster springs摇枕弹簧damper减震器阻尼器longitudinalanti-yawing dampers纵向抗蛇行减震器disc brakes 盘形制动traction transfer device 牵引装置wheel tread brakes踏面制动tread gradient踏面斜self-steering自导向Vehicle Suspension车辆悬挂cushion system缓冲装置vertical movement垂向振动primary suspension一系悬挂装secondary suspension 二系悬挂装置车钩coupler摇枕bolster乘务员crew轴箱axlebox棚车boxcar封闭车housing car保温refrigerator car牲畜车stock car漏斗车hopper罐车tank car集装箱container车体carboy复合车combine car邮政车railway post office圆顶车dome转向架bogie瞭望车observation car缓冲器draft gear行李车baggage car卧铺车sleeping car旁承side bearer制动缸brake cylinder侧梁side beams横梁cross beamscrosstie敞车gondola 英译汉1.The basic design of apassenger car hasn”t changed muchsince the middle of the 19thcentury,but there are severaldifferent passenger car types inservice around the world.2.自19世纪中期以来,客车的基本设计没有发生多大改变,但仍有不同形式的客车在全世界范围内使用。
汽车设计师的英文单词
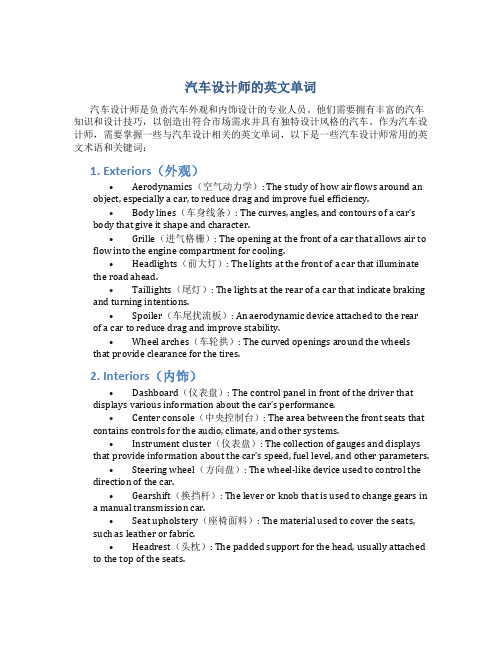
汽车设计师的英文单词汽车设计师是负责汽车外观和内饰设计的专业人员。
他们需要拥有丰富的汽车知识和设计技巧,以创造出符合市场需求并具有独特设计风格的汽车。
作为汽车设计师,需要掌握一些与汽车设计相关的英文单词,以下是一些汽车设计师常用的英文术语和关键词:1. Exteriors(外观)•Aerodynamics(空气动力学): The study of how air flows around an object, especially a car, to reduce drag and improve fuel efficiency.•Body lines(车身线条): The curves, angles, and contours of a car’s body that give it shape and character.•Grille(进气格栅): The opening at the front of a car that allows air to flow into the engine compartment for cooling.•Headlights(前大灯): The lights at the front of a car that illuminate the road ahead.•Taillights(尾灯): The lights at the rear of a car that indicate braking and turning intentions.•Spoiler(车尾扰流板): An aerodynamic device attached to the rear of a car to reduce drag and improve stability.•Wheel arches(车轮拱): The curved openings around the wheels that provide clearance for the tires.2. Interiors(内饰)•Dashboard(仪表盘): The control panel in front of the driver that displays various information about the car’s performance.•Center console(中央控制台): The area between the front seats that contains controls for the audio, climate, and other systems.•Instrument cluster(仪表盘): The collection of gauges and displays that provide information about the car’s speed, fuel level, and other parameters.•Steering wheel(方向盘): The wheel-like device used to control the direction of the car.•Gearshift(换挡杆): The lever or knob that is used to change gears ina manual transmission car.•Seat upholstery(座椅面料): The material used to cover the seats, such as leather or fabric.•Headrest(头枕): The padded support for the head, usually attached to the top of the seats.3. Materials(材料)•Carbon fiber(碳纤维): A lightweight yet strong material used in the construction of high-performance cars.•Aluminum(铝): A lightweight and corrosion-resistant metal used in various parts of a car, such as the body panels and engine components.•Leather(皮革): A durable and luxurious material often used to cover the seats and steering wheel.•Plastic(塑料): A versatile and lightweight material used in many interior and exterior components of a car.•Fabric(织物): A textile material used for seat upholstery and other soft surfaces in a car’s interior.4. Production(生产)•Design sketch(设计草图): A hand-drawn or computer-generated drawing that shows the initial concept or idea for a car’s design.•Clay model(粘土模型): A three-dimensional representation of a car’s design, often used in the early stages of the desig n process.•CAD (Computer-Aided Design)(计算机辅助设计): The use of computer software to create precise and detailed 2D and 3D models of a car’s design.•Prototype(原型): A working model of a car that is built to test and evaluate its design and performance before mass production.•Assembly line(生产线): A series of workstations where different components of a car are assembled together to create the final product.•Quality control(质量控制): The processes and procedures used to ensure that each car meets the required standards of quality and safety.以上是一些汽车设计师常用的英文单词和术语。
设计车辆的方案英文缩写
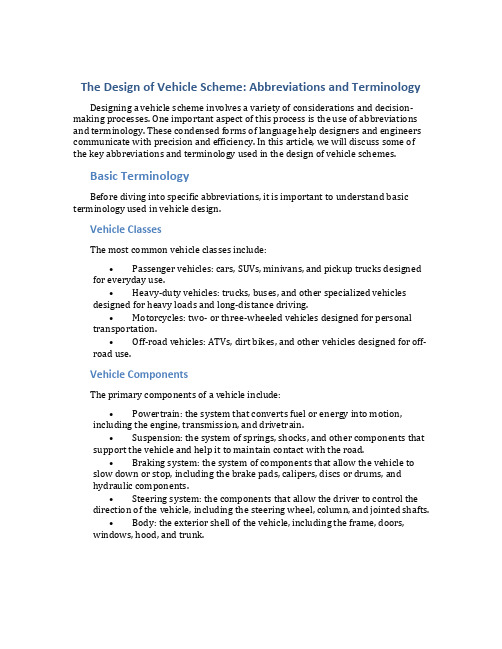
The Design of Vehicle Scheme: Abbreviations and Terminology Designing a vehicle scheme involves a variety of considerations and decision-making processes. One important aspect of this process is the use of abbreviations and terminology. These condensed forms of language help designers and engineers communicate with precision and efficiency. In this article, we will discuss some of the key abbreviations and terminology used in the design of vehicle schemes.Basic TerminologyBefore diving into specific abbreviations, it is important to understand basic terminology used in vehicle design.Vehicle ClassesThe most common vehicle classes include:•Passenger vehicles: cars, SUVs, minivans, and pickup trucks designed for everyday use.•Heavy-duty vehicles: trucks, buses, and other specialized vehicles designed for heavy loads and long-distance driving.•Motorcycles: two- or three-wheeled vehicles designed for personal transportation.•Off-road vehicles: ATVs, dirt bikes, and other vehicles designed for off-road use.Vehicle ComponentsThe primary components of a vehicle include:•Powertrain: the system that converts fuel or energy into motion, including the engine, transmission, and drivetrain.•Suspension: the system of springs, shocks, and other components that support the vehicle and help it to maintain contact with the road.•Braking system: the system of components that allow the vehicle to slow down or stop, including the brake pads, calipers, discs or drums, andhydraulic components.•Steering system: the components that allow the driver to control the direction of the vehicle, including the steering wheel, column, and jointed shafts.•Body: the exterior shell of the vehicle, including the frame, doors, windows, hood, and trunk.Common AbbreviationsNow that we have a basic understanding of vehicle design terminology, let’s explore common abbreviations used in the design of vehicle schemes.CAEComputer-Aided Engineering (CAE) is a method of using computer software to simulate and analyze the behavior of a vehicle in various conditions. This process can help designers optimize the performance, safety, and efficiency of their schemes.CADComputer-Aided Design (CAD) is a process of using specialized software to create detailed 2D or 3D models of vehicle components. CAD software can help designers visualize and test their designs before creating physical prototypes.CFDComputational Fluid Dynamics (CFD) is a type of CAE technique that uses mathematical simulations to predict the behavior of fluids (such as air or liquids) in various conditions. This technique is often used to optimize the aerodynamics of a vehicle.DFMDesign for Manufacturability (DFM) is a process of designing vehicle components with the goal of optimizing their manufacturability. DFM techniques can help minimize production costs, improve the consistency and quality of the final product, and reduce the risk of defects or errors.HVACHeating, Ventilation, and Air Conditioning (HVAC) is the system of components that regulates the temperature and air quality inside a vehicle. HVAC systems can include components such as air filters, fans, ducts, and refrigerant.IHSInflatable Headrest System (IHS) is an innovative safety feature that has been developed by some automakers. This system deploys a set of airbags inside the headrests of the front seats to protect occupants in the event of a rear-end collision.MPGMiles per Gallon (MPG) is a measurement of a vehicle’s fuel efficiency. This metric indicates how far the vehicle can travel on a gallon of fuel.OEMOriginal Equipment Manufacturer (OEM) refers to companies that manufacture parts and components that are used in the production of vehicles. OEM parts are designed to meet the original specifications of the vehicle manufacturer, and are often considered to be of higher quality and reliability than aftermarket parts.PHEVPlug-In Hybrid Electric Vehicle (PHEV) is a type of hybrid vehicle that can be charged by plugging it into an electrical outlet. PHEVs typically have larger batteries than conventional hybrid vehicles and can drive for longer distances on electricity alone.RWDRear-Wheel Drive (RWD) is a type of drivetrain layout in which power is transmitted to the rear wheels of the vehicle. RWD vehicles are often favored by performance enthusiasts for their balance and handling characteristics.TPMSTire Pressure Monitoring System (TPMS) is a safety feature that alerts drivers when the pressure in one or more tires is too low. TPMS systems can help improve fuel efficiency, reduce tire wear, and prevent accidents caused by tire blowouts.ConclusionDesigning a vehicle scheme involves a complex array of considerations, and language plays a critical role in facilitating effective communication and decision-making processes. Abbreviations and terminology help designers and engineers to communicate with precision and efficiency, ultimately leading to better-designed vehicle schemes. By understanding the most common abbreviations and terminology used in vehicle design, you can gain a deeper understanding of this fascinating field and appreciate the innovation and complexity that goes into each vehicle on the road today.。
想象中的超级跑鞋的英语作文

想象中的超级跑鞋的英语作文The Paragon of Performance: A Visionary Conception of the Ultimate Supercar.In the realm of automotive engineering, the pursuit of perfection drives countless minds to envision machines that transcend the boundaries of conventional perception. The supercar, a testament to this unyielding aspiration, embodies the pinnacle of automotive excellence, pushing the limits of speed, agility, and aesthetics. Yet, even within this extraordinary echelon, there exists an unfulfilled yearning for a vehicle that transcends current capabilities, a machine that represents the zenith of human ingenuity and technological prowess.Behold, the Supercar of Tomorrow, a visionary creation that embodies the essence of automotive supremacy. It is a symphony of cutting-edge technologies and innovative design principles, meticulously orchestrated to deliver an unparalleled driving experience that defies the limits ofthe imagination.Aerodynamic Prowess.The Supercar of Tomorrow seamlessly harnesses the forces of nature to achieve unparalleled aerodynamic efficiency. Its sleek silhouette is meticulously sculpted, with every curve and contour engineered to minimize drag and maximize stability. Activeаэродинамические системы, including adjustable spoilers and diffusers, dynamically adjust to optimize downforce and enhance handling prowess. The result is a vehicle that glides through the air with effortless grace, its aerodynamic prowess enabling blistering speeds and razor-sharp cornering.Propulsion Beyond Compare.At the heart of the Supercar of Tomorrow lies a powertrain that redefines the very concept of automotive performance. Hybrid technology seamlessly integrates the explosive power of a high-performance internal combustionengine with the instantaneous torque of an electric motor, generating a symphony of relentless acceleration. Advanced energy recovery systems capture every ounce of kinetic energy, ensuring maximum efficiency and extended range. The result is a powertrain that delivers staggering performance, propelling the Supercar of Tomorrow from 0 to 60 miles per hour in a heart-stopping matter of seconds and reaching breathtaking top speeds that challenge the boundaries of physics.Precision Handling and Control.Absolute control is paramount in the Supercar of Tomorrow. Its chassis is a masterpiece of engineering, meticulously crafted from ultra-lightweight materials that provide exceptional rigidity and torsional strength. Advanced suspension systems, featuring electronically controlled dampers and active anti-roll bars, ensure that the vehicle remains planted firmly on the road, providing unparalleled handling precision and stability. The steering system, with its variable ratios and haptic feedback, delivers an intuitive and responsive connection between thedriver and the machine.A Cockpit of Innovation.The interior of the Supercar of Tomorrow is a sanctuary of technological sophistication and ergonomic excellence. The driver is enveloped in a cocoon of comfort, surrounded by a fully digital cockpit that seamlessly integratescutting-edge infotainment, navigation, and vehicle performance data. Advanced head-up display systems project vital information directly onto the windshield, keeping the driver's focus firmly on the road ahead. Ambient lighting and advanced sound systems create an immersive and stimulating environment that enhances the drivingexperience to new heights.Intelligent Assistance.The Supercar of Tomorrow transcends the boundaries of mere transportation, embracing the latest advancements in artificial intelligence and autonomous driving technologies. Intelligent systems monitor the vehicle's surroundings,providing real-time assistance and enhancing safety. Adaptive cruise control adjusts speed and braking to maintain a safe distance from other vehicles. Lane departure warning systems and blind spot monitoring alert the driver to potential hazards, reducing the risk of accidents. The Supercar of Tomorrow is a partner on the road, offering peace of mind and enhancing the overall driving experience.A Vision of the Future.The Supercar of Tomorrow is not merely a concept; it is a glimpse into the future of automotive performance. It is a testament to the ingenuity and ambition of engineers and designers who refuse to accept the limitations of the present. As technology continues to evolve, the Supercar of Tomorrow will undoubtedly surpass even these lofty aspirations, becoming an icon of automotive excellence that inspires generations to come.For now, it remains a tantalizing vision, a beacon of innovation that drives us forward in the relentless pursuitof automotive perfection. The Supercar of Tomorrow is the embodiment of our dreams, a machine that transcends the boundaries of possibility and redefines the very essence of driving.。
新能源汽车英语名词练习40题
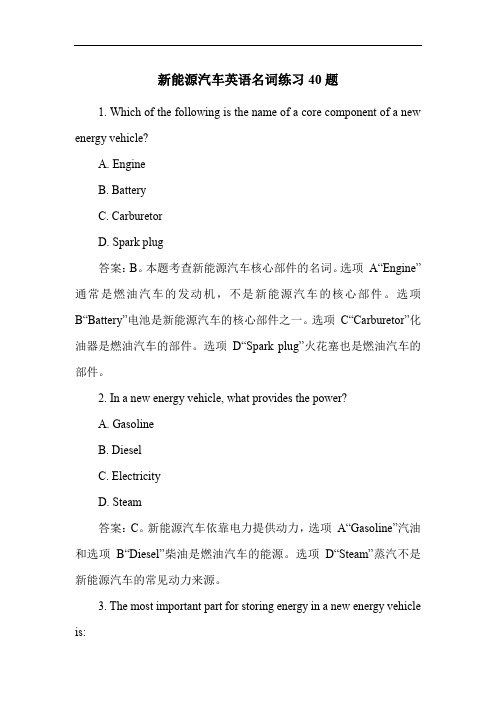
新能源汽车英语名词练习40题1. Which of the following is the name of a core component of a new energy vehicle?A. EngineB. BatteryC. CarburetorD. Spark plug答案:B。
本题考查新能源汽车核心部件的名词。
选项A“Engine”通常是燃油汽车的发动机,不是新能源汽车的核心部件。
选项B“Battery”电池是新能源汽车的核心部件之一。
选项C“Carburetor”化油器是燃油汽车的部件。
选项D“Spark plug”火花塞也是燃油汽车的部件。
2. In a new energy vehicle, what provides the power?A. GasolineB. DieselC. ElectricityD. Steam答案:C。
新能源汽车依靠电力提供动力,选项A“Gasoline”汽油和选项B“Diesel”柴油是燃油汽车的能源。
选项D“Steam”蒸汽不是新能源汽车的常见动力来源。
3. The most important part for storing energy in a new energy vehicle is:A. Fuel tankB. Battery packC. Exhaust pipeD. Radiator答案:B。
选项A“Fuel tank”油箱是燃油汽车存储燃料的地方。
选项B“Battery pack”电池组用于存储新能源汽车的电能。
选项C“Exhaust pipe”排气管用于排放尾气,不是存储能量的部件。
选项D“Radiator”散热器用于散热。
4. Which one is not a common core component of a new energy vehicle?A. MotorB. TransmissionC. AlternatorD. Inverter答案:C。
2024北京石景山高三一模英语(教师版)
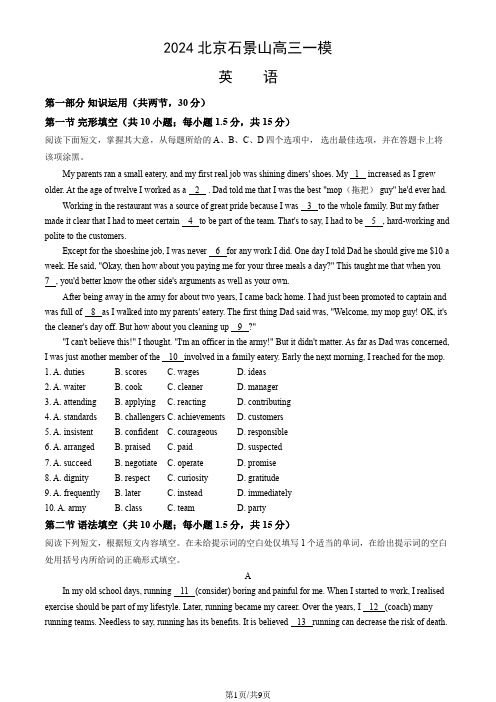
2024北京石景山高三一模英语第一部分知识运用(共两节,30分)第一节完形填空(共10小题;每小题1.5分,共15分)阅读下面短文,掌握其大意,从每题所给的A、B、C、D四个选项中,选出最佳选项,并在答题卡上将该项涂黑。
My parents ran a small eatery, and my first real job was shining diners' shoes. My 1 increased as I grew older. At the age of twelve I worked as a 2 . Dad told me that I was the best "mop(拖把) guy" he'd ever had.Working in the restaurant was a source of great pride because I was 3 to the whole family. But my father made it clear that I had to meet certain 4 to be part of the team. That's to say, I had to be 5 , hard-working and polite to the customers.Except for the shoeshine job, I was never 6 for any work I did. One day I told Dad he should give me $10 a week. He said, "Okay, then how about you paying me for your three meals a day?" This taught me that when you 7 , you'd better know the other side's arguments as well as your own.After being away in the army for about two years, I came back home. I had just been promoted to captain and was full of 8 as I walked into my parents' eatery. The first thing Dad said was, "Welcome, my mop guy! OK, it's the cleaner's day off. But how about you cleaning up 9 ?""I can't believe this!" I thought. "I'm an officer in the army!" But it didn't matter. As far as Dad was concerned,I was just another member of the 10 involved in a family eatery. Early the next morning, I reached for the mop.1. A. duties B. scores D. ideas2. A. waiter B. cook C. cleaner D. manager3. A. attending B. applying C. reacting D. contributing4. A. standards B. challengers C. achievements D. customers5. A. insistent B. confident C. courageous D. responsible6. A. arranged B. praised C. paid D. suspected7. A. succeed B. negotiate C. operate D. promise8. A. dignity B. respect C. curiosity D. gratitude9. A. frequently B. later C. instead D. immediately10. A. army B. class C. team D. party第二节语法填空(共10小题;每小题1.5分,共15分)阅读下列短文,根据短文内容填空。
学术英语习题及部分答案
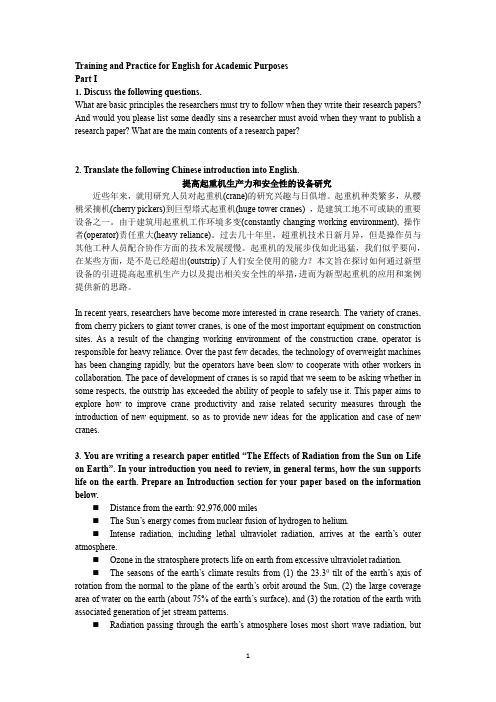
Training and Practice for English for Academic PurposesPart I1.Discuss the following questions.What are basic principles the researchers must try to follow when they write their research papers? And would you please list some deadly sins a researcher must avoid when they want to publish a research paper? What are the main contents of a research paper?2. Translate the following Chinese introduction into English.提高起重机生产力和安全性的设备研究近些年来,就用研究人员对起重机(crane)的研究兴趣与日俱增。
起重机种类繁多,从樱桃采摘机(cherry pickers)到巨型塔式起重机(huge tower cranes) ,是建筑工地不可或缺的重要设备之一。
由于建筑用起重机工作环境多变(constantly changing working environment), 操作者(operator)责任重大(heavy reliance)。
过去几十年里,超重机技术日新月异,但是操作员与其他工种人员配合协作方面的技术发展缓慢。
起重机的发展步伐如此迅猛,我们似乎要问,在某些方面,是不是已经超出(outstrip)了人们安全使用的能力?本文旨在探讨如何通过新型设备的引进提高起重机生产力以及提出相关安全性的举措,进而为新型起重机的应用和案例提供新的思路。
In recent years, researchers have become more interested in crane research.The variety of cranes, from cherry pickers to giant tower cranes, is one of the most important equipment on construction sites.As a result of the changing working environment of the construction crane, operator is responsible for heavy reliance.Over the past few decades, the technology of overweight machines has been changing rapidly, but the operators have been slow to cooperate with other workers in collaboration.The pace of development of cranes is so rapid that we seem to be asking whether in some respects, the outstrip has exceeded the ability of people to safely use it.This paper aims to explore how to improve crane productivity and raise related security measures through the introduction of new equipment, so as to provide new ideas for the application and case of new cranes.3. You are writing a research paper entitled “The Effects of Radiation from the Sun on Life o n Earth”. In your introduction you need to review, in general terms, how the sun supports life on the earth. Prepare an Introduction section for your paper based on the information below.⏹Distance from the earth: 92,976,000 miles⏹The Sun’s energy comes from nuclear fusion of hydrogen to helium.⏹Intense radiation, including lethal ultraviolet radiation, arrives at the earth’s outer atmosphere.⏹Ozone in the stratosphere protects life on earth from excessive ultraviolet radiation.⏹The seasons of the earth’s climate results from (1) the 23.30tilt of the earth’s axis of rotation from the normal to the plane of the earth’s orbit around the Sun, (2) the large coverage area of water on the earth (about 75% of the earth’s surface), an d (3) the rotation of the earth with associated generation of jet-stream patterns.⏹Radiation passing through the earth’s atmosphere loses most short-wave radiation, butsome arriving at the surface is converted into infrared radiation which is then trapped by water vapor and other tri-atomic molecules in the troposphere and stratosphere, causing global warming.Life on earth is maintained from photosynthesis and conversion of carbon dioxide to oxygen by plants.4.Translate the following parts of sentences in Introduction into proper English.(1)过去对……的研究工作说明……The previous work on … has indicated that…(2)A在1932年做了关于……的早期研究。
兰州交通大学车辆工程专业毕业论文 30t轴重货车转向架摇枕的结构及设计

摘要随着我国铁路运输向高速和重载方向发展,转向架摇枕的运用条件日趋苛刻。
因此,研究重载运输条件下的摇枕结构及其性能变得尤为重要。
本文通过国内外重载运输的发展现状的了解,分析了国内外现有重载货车转向架的结构特点和性能。
首先对我国30t轴重转向架进行了结构选型,初步确定了适应我国国情的30t轴重货车转向架的基本结构型式。
重点对该转向架的摇枕的结构进行自主设计与分析。
运用SolidWorks建立30t轴重货车转向架摇枕的三维模型,并且详细介绍了该转向架摇枕的结构特点及设计步骤,其中包括了外壁和内部筋板厚度、排水口、漏沙孔等工艺孔、摇枕斜楔、15旁承台座、16弹簧承台、心盘部位、摇枕吊耳。
然后对该摇枕进行了有限元应力分析,然后根据分析结果确定摇枕的薄弱地方并进行改进,最终设计得到满足强度要求,并有足够抗疲劳性能的转向架摇枕。
关键词:重载货车;转向架;摇枕;设计;有限元分析AbstractWith the development of railway transportation in China to high speed and heavy, truck bolster conditions using the increasingly harsh.Therefore, studying under heavy haul bolster structure and performance has become particularly important. The current development status at home and abroad through the heavy haul transportation understanding, analysis of the structure characteristics and performance of the existing heavy truck frame at home and abroad to .The first of China's 30t axle load bogie structure selection, initially identified the basic structure frame of the axle load 30t China steering. The focus of the steering structure bolster frame of independent design and analysis.Use SolidWorks to set up 30t axle load truck steering frame bolster 3D model, and introduces the structure characteristics and design steps of steering frame bolster, including the outer and inner rib plate thickness, drains, drain sand hole technology of hole, bolster wedge, a side bearing pedestal, spring seat, center plate position, swing hanger lug. Then the bolster the finite element stress analysis, then bolster weak place and determined according to the analysis results, the final design can meet the strength requirements, and has enough fatigue performance of the bogie bolster.Key words: heavy-duty freight car; Bogie;Bogie Bolster; Design;The finite element analysis目录1 绪论------------------------------------------------------------------------------------------------- 11.1 选题背景---------------------------------------------------------------------------------- 11.2 国外重载车辆的现状------------------------------------------------------------------- 11.3 我国近年来重载车辆现状------------------------------------------------------------- 21.4 本文主要工作---------------------------------------------------------------------------- 22 国内外大轴重转向架的现状------------------------------------------------------------------- 32.1 国外大轴重转向架---------------------------------------------------------------------- 32.2 我国国内及出口大轴重转向架 ------------------------------------------------------ 53 转向架的结构选型------------------------------------------------------------------------------- 83.1 转向架选型原则------------------------------------------------------------------------- 83.2 转向架结构特点------------------------------------------------------------------------- 84 转向架摇枕的设计------------------------------------------------------------------------------104.1 大轴重转向架载重分析和损伤部位探究 -----------------------------------------104.2 转向架摇枕的三维结构设计 --------------------------------------------------------124.2.1 总体结构设计------------------------------------------------------------------124.2.2 外壁和内部筋板厚度的设计 -----------------------------------------------134.2.3 排水口、漏沙孔等工艺孔的设计 -----------------------------------------144.2.4 摇枕斜楔的设计---------------------------------------------------------------154.2.5 旁承台座的设计---------------------------------------------------------------164.2.6 弹簧承台的设计---------------------------------------------------------------174.2.7 心盘部位的设计---------------------------------------------------------------174.2.8 摇枕吊耳的设计---------------------------------------------------------------184.2.9 棱角的圆弧过渡设计 --------------------------------------------------------195 转向架摇枕的有限元分析---------------------------------------------------------------------215.1.1 有限元求解问题的思路及方法 --------------------------------------------215.1.2 转向架摇枕承受载荷情况分析 --------------------------------------------225.1.3 转向架摇枕使用Simulation进行有限元分析---------------------------235.1.4 转向架改进措施---------------------------------------------------------------275.1.5 本章小结------------------------------------------------------------------------27结论 ---------------------------------------------------------------------------------------------------28 致谢 ---------------------------------------------------------------------------------------------------29 参考文献---------------------------------------------------------------------------------------------301 绪论1.1 选题背景我们国家自从改革开放到现在,随着国民经济的一直深入的发展和人民生活水平的显著提高,我们对货物运输效率的要求也是越来越高了。
剪叉式高空车转向机构结构分析

position determination and force relationship of steering mechanism are solved, which can provide theoretical basis for the
design and optimization of steering mechanism of AWP.
Keywords: aerial work platform; steering mechanism; steering resistance moment; analysis
0引言
所示,角度参数在引用时指明含义。
转向机构是高空作业车转向系统的主要执行组件, 为适应高空车在狭小的空间移动转向等工况,需要转向 机构能够使作业车有较小的转弯半径,同时尽可能减轻 或避免磨胎,对转向机构的研究有利于增强高空车的转 向性能、改善磨胎、减小转向力和增强操作稳定性。
Abstract: Steering mechanism is the executive component of steering system of aerial work platform(AWP). The selection
and design of steering mechanism determine the steering performance of working vehicle. The design of steering
机械工程师
MECHANICAL ENGINEER
剪叉式高空车转向机构结构分析
陆进添, 刘丹, 邓安田 (三一帕尔菲格特种车辆装备有限公司 研究院,江苏 南通 226000)
摘 要:转向机构是高空作业车转向系统的执行组件,转向机构的选择和设计决定了作业车的转向性能。而转向机构的设
英语作文-体验快乐驾驶,驾驶乐趣无限
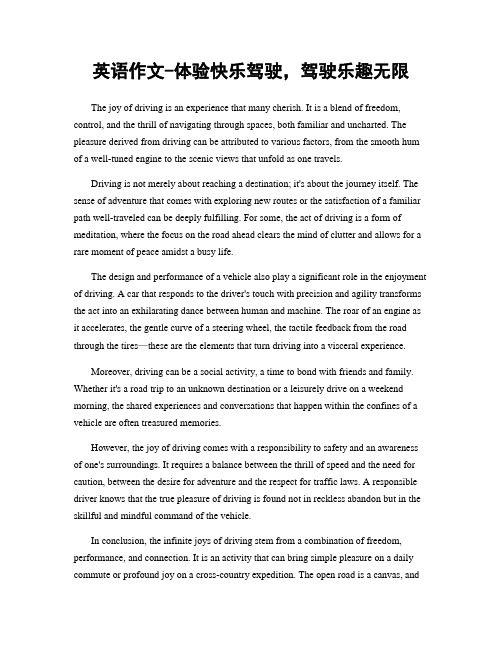
英语作文-体验快乐驾驶,驾驶乐趣无限The joy of driving is an experience that many cherish. It is a blend of freedom, control, and the thrill of navigating through spaces, both familiar and uncharted. The pleasure derived from driving can be attributed to various factors, from the smooth hum of a well-tuned engine to the scenic views that unfold as one travels.Driving is not merely about reaching a destination; it's about the journey itself. The sense of adventure that comes with exploring new routes or the satisfaction of a familiar path well-traveled can be deeply fulfilling. For some, the act of driving is a form of meditation, where the focus on the road ahead clears the mind of clutter and allows for a rare moment of peace amidst a busy life.The design and performance of a vehicle also play a significant role in the enjoyment of driving. A car that responds to the driver's touch with precision and agility transforms the act into an exhilarating dance between human and machine. The roar of an engine as it accelerates, the gentle curve of a steering wheel, the tactile feedback from the road through the tires—these are the elements that turn driving into a visceral experience.Moreover, driving can be a social activity, a time to bond with friends and family. Whether it's a road trip to an unknown destination or a leisurely drive on a weekend morning, the shared experiences and conversations that happen within the confines of a vehicle are often treasured memories.However, the joy of driving comes with a responsibility to safety and an awareness of one's surroundings. It requires a balance between the thrill of speed and the need for caution, between the desire for adventure and the respect for traffic laws. A responsible driver knows that the true pleasure of driving is found not in reckless abandon but in the skillful and mindful command of the vehicle.In conclusion, the infinite joys of driving stem from a combination of freedom, performance, and connection. It is an activity that can bring simple pleasure on a daily commute or profound joy on a cross-country expedition. The open road is a canvas, andthe vehicle, a brush with which one can paint experiences that are as varied as they are delightful. The happiness that comes from driving is as limitless as the roads one can explore. 。
FURUNO FEA-2x07 自动驾驶系统操作手册说明书
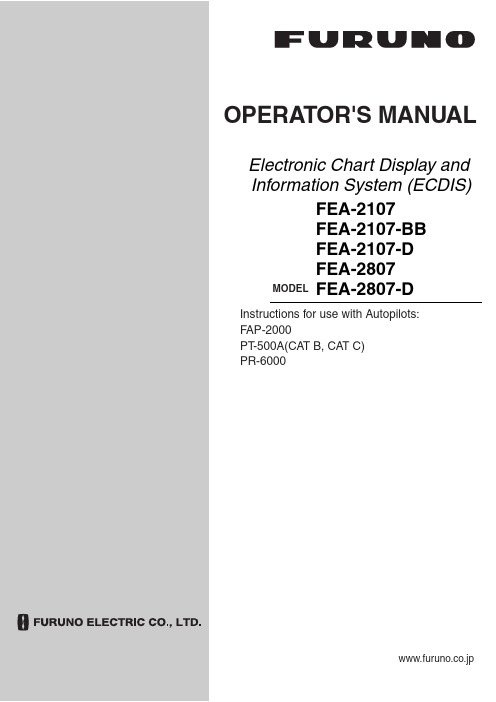
Electronic Chart Display and Information System (ECDIS)OPERATOR'S MANUALwww.furuno.co.jpMODEL FEA-2107 FEA-2107-BB FEA-2107-D FEA-2807FEA-2807-DInstructions for use with Autopilots: FAP-2000PT -500A(CAT B, CAT C) PR-6000The paper used in this manualis elemental chlorine free.9-52 Ashihara-cho,Nishinomiya, 662-8580, JAPANForewordThe purpose of this manual is to provide the instructions for how to use the Autopilots FAP-2000,PT-500A(CAT B and CAT C)1 and PR-60002 with the FURUNO FEA-2107-D, FEA-2107-BB-D, FEA-2807-D Electronic Chart Display and Information System (ECDIS). For ECDIS operating information, see its Operator's Manual.1 PT-500A(CAT B and CAT C) are products of YOKOGAWA ELECTRIC CORPORATION.2 PR-6000 is a product of TOKYO KEIKI INCImportant Notices• The operator of this equipment must read and follow the descriptions in this manual. Wrong operation can cancel the warranty or cause injury.• Do not copy any part of this manual without written permission from FURUNO.• If this manual is lost or worn, contact your dealer about replacement.• The contents of this manual and equipment specifications can change without notice.• The example screens (or illustrations) shown in this manual can be different from the screens you see on your display. The screens you see depend on your system configuration and equipment settings.• Save this manual for future reference.• Any modification of the equipment (including software) by persons not authorized by FURUNO will cancel the warranty.• All brand and product names are trademarks, registered trademarks or service marks of their respective holders.iSafety Instructionsiiabout replacement.ype: 86-003-1011-0Safety InstructionsTable of ContentsSYSTEM CONFIGURATION (vii)1.FURUNO Autopilot FAP-2000..............................................................................................1-11.1Introduction.............................................................................................................................1-11.2Control Panel...........................................................................................................................1-11.3Steering Modes........................................................................................................................1-31.3.1Hand steering modes..................................................................................................1-31.3.2Autopilot steering modes............................................................................................1-31.3.3Route steering modes..................................................................................................1-51.4Additional Information About Steering Modes....................................................................1-161.5Important Information About Steering Mode Changes......................................................1-181.6Autopilot Display in Sidebar.................................................................................................1-191.7Expected Steering Performance Under Various Conditions...............................................1-201.7.1Expected steering performance for going ahead.....................................................1-201.7.2Expected steering performance for turns................................................................1-231.8Expected Steering Performance Under Critical Failure.....................................................1-251.8.1Lost heading from autopilot (ECDIS may also have lost heading)........................1-251.8.2Lost heading from ECDIS (autopilot still has heading).........................................1-251.8.3Lost speed..................................................................................................................1-261.8.4Low speed..................................................................................................................1-261.8.5Lost SOG/COG reference..........................................................................................1-271.8.6Total lost position......................................................................................................1-281.8.7Lost differential position..........................................................................................1-291.8.8Lost differential position and position discrepancy................................................1-301.8.9Lost communication between ECDIS and autopilot...............................................1-301.8.10Lost communication between autopilot and ECDIS...............................................1-301.9Other Operations...................................................................................................................1-311.10Alerts......................................................................................................................................1-311.10.1Alerts generated by autopilot...................................................................................1-311.10.2Alerts generated by ECDIS......................................................................................1-321.10.3Error alerts generated by autopilot.........................................................................1-371.11How to Use the Curved EBL.................................................................................................1-381.11.1What is the curved EBL?..........................................................................................1-381.11.2Structure of the curved EBL.....................................................................................1-391.11.3How to design a new turn while the ship is turning...............................................1-391.12How to Use the Predictor......................................................................................................1-402.YOKOGAWA Autopilot PT-500A (category B).......................................................................2-12.1Introduction.............................................................................................................................2-12.2Steering Control Unit..............................................................................................................2-12.3Steering Modes........................................................................................................................2-22.3.1Hand steering mode (Mode selector: HAND)............................................................2-22.3.2Autopilot steering mode (Mode selector: AUTO).......................................................2-22.3.3Route steering mode (Mode selector: NAVI)..............................................................2-22.4Other Operations...................................................................................................................2-102.5Alerts Generated by ECDIS..................................................................................................2-102.5.1Operational alerts.....................................................................................................2-102.5.2Error alerts................................................................................................................2-132.6Autopilot Display in Sidebar.................................................................................................2-142.7Expected Steering Performance Under Various Conditions...............................................2-152.7.1Expected steering performance for going ahead.....................................................2-152.7.2Expected steering performance for turns................................................................2-162.8Expected Steering Performance Under Critical Failure.....................................................2-172.8.1Lost heading from autopilot (ECDIS may also have lost heading)........................2-172.8.2Lost heading from ECDIS (autopilot still has heading).........................................2-17iiiTable of Contentsiv2.8.3Lost speed..................................................................................................................2-182.8.4Low speed..................................................................................................................2-192.8.5Total lost position......................................................................................................2-202.8.6Lost differential position and position discrepancy................................................2-212.8.7Lost communication between ECDIS and autopilot...............................................2-222.8.8Lost communication between autopilot and ECDIS...............................................2-22 2.9How to Use the Curved EBL.................................................................................................2-23 2.10How to Use the Predictor......................................................................................................2-243.YOKOGAWA Autopilot PT-500A (category C).......................................................................3-13.1Introduction..............................................................................................................................3-13.2Steering Control Unit..............................................................................................................3-13.3Steering Modes.........................................................................................................................3-23.3.1Hand steering mode (Mode selector: HAND)............................................................3-23.3.2Autopilot steering mode (Mode selector: AUTO).......................................................3-23.3.3Route steering mode (Mode selector: NAVI)..............................................................3-23.4Other Operations...................................................................................................................3-113.5Alerts Generated by ECDIS..................................................................................................3-123.5.1Operational alerts.....................................................................................................3-123.5.2Error alerts................................................................................................................3-123.6Autopilot Display in Sidebar.................................................................................................3-133.7Expected Steering Performance Under Various Conditions...............................................3-143.7.1Expected steering performance for going ahead.....................................................3-143.7.2Expected steering performance for turns................................................................3-153.8Expected Steering Performance Under Critical Failure.....................................................3-163.8.1Lost heading from autopilot (ECDIS may also have lost heading)........................3-163.8.2Lost heading from ECDIS (autopilot still has heading).........................................3-163.8.3Lost speed..................................................................................................................3-173.8.4Low speed..................................................................................................................3-173.8.5Lost SOG/COG reference in GoAW mode................................................................3-183.8.6Total lost position......................................................................................................3-183.8.7Lost differential position in GoAW mode.................................................................3-193.8.8Lost differential position and position discrepancy................................................3-203.8.9Lost communication between ECDIS and autopilot...............................................3-213.8.10Lost communication between autopilot and ECDIS...............................................3-213.9How to Use the Curved EBL.................................................................................................3-214.TOKYO KEIKI Autopilot PR-6000.......................................................................................4-14.1Introduction..............................................................................................................................4-14.2Steering Control Unit..............................................................................................................4-14.3Steering Modes.........................................................................................................................4-24.3.1Hand steering mode (Mode selector: HAND)............................................................4-24.3.2Autopilot steering mode (Mode selector: AUTO).......................................................4-34.3.3Non-Follow-Up steering mode (Mode selector: NFU)...............................................4-34.3.4Remote hand steering mode (Mode selector: RC)......................................................4-44.3.5Steering override units...............................................................................................4-54.3.6Route steering mode, RC/Nav (Mode: RC).................................................................4-64.4HCS Unit Controls.................................................................................................................4-174.5Alerts Generated by ECDIS..................................................................................................4-184.5.1Operational alerts.....................................................................................................4-184.5.2Error alerts................................................................................................................4-184.6Autopilot Display in Sidebar.................................................................................................4-194.7Expected Steering Performance Under Various Conditions...............................................4-214.7.1Expected steering performance for going ahead.....................................................4-214.7.2Expected steering performance for turns................................................................4-224.8Expected Steering Performance Under Critical Failure.....................................................4-244.8.1Lost heading from autopilot (ECDIS may also have lost heading).......................4-244.8.2Lost heading from ECDIS (autopilot still has heading).........................................4-244.8.3Lost speed..................................................................................................................4-254.8.4Low speed..................................................................................................................4-25Table of Contentsv4.8.5 Lost SOG/COG reference in GoAW steering mode .................................................4-26 4.8.6 Total lost position......................................................................................................4-26 4.8.7 Lost differential position in GoAW steering mode..................................................4-27 4.8.8 Lost differential position and position discrepancy................................................4-27 4.8.9 Lost communication between ECDIS and autopilot...............................................4-28 4.8.10 Lost communication between autopilot and ECDIS...............................................4-28 4.9 How to Use the Curved EBL.................................................................................................4-29 4.10 How to Use the Predictor......................................................................................................4-30Index..........................................................................................................................................IN-1Table of ContentsThis page intentionally left blank. viSYSTEM CONFIGURATIONSingle workstationviiSystem Configurationviii Multi workstation: M A N D A T O R Y: A L T E R N A T I V E : O P T I O N A L : O P T I O N A L , B U T N O T U S E D B Y T C S : E X T E R N A L S E N S O R o r E Q U I P M E N TN o t e 1 A l t e r n a t i v e s a r e , G y r o c o m p a s s b y I E C 61162 o r S t e p p e rN o t e 2 A l t e r n a t i v e s a r e , L o g b y I E C 61162 o r 200p /n m1. FURUNO AutopilotFAP-20001.1 IntroductionThis chapter describes the steering functions available with the FURUNO Autopilot FAP-2000.1.2 ControlPanelThis section describes the FAP-2000's control panel.FURUNO1-6: Steering mode selection• PROGRAM TRACK:Program Track-controlled heading change using set radius. Also for steering with selected TT models.• GOTO TRACK:Track-controlled route steering• GOTO WPT: Course-controlled route steering• HEADING CONTROL: Immediate heading-controlled course change using set rudder angle limit• RADIUS CONTROL: Immediate radius-controlled course change using set radius• PROGRAM HEADING CHANGE: Program heading-controlled course change using set radius1-11. FURUNO Autopilot FAP-20007: Loading condition indicator: Loading conditions, Light, Medium or Loaded8: Performance indicator:Performance status, Economy, Medium or Precise9: Status indicator: Shows selected mode and state of readiness:• FAP-2000 in operation: READY• FAP-2000 control mode: HEADING/TRACK• FAP-2000 route steering: ROUTE• FAP-2000 track-controlled turn: TURN10: Alert indicator and buzzer cancel• ALERT lamp for other FAP-2000-related alerts and errors.• RESET button for acknowledging other FAP-2000-related alerts and errors.11: Special function keypads• Manually input speed.• Adjust panel dimmer.• Set manual speed value, auto speed, rudder limit function, performance and conditions.12: Tiller• Set course and radius.• PORT and STBD lamps show when the tiller can be used to set course.• INCREASE. and DECREASE. lamps show when the tiller can be used to set a radius or a rudder angle limit. 13: Gyro reading and a bar graph showing rate of turn14: Set Heading display includes:• Off course alert• Turn side• Rudder-on limit indication15: Rudder displays:• Radius set-point in the Radius Control mode• Rudder limit in the Heading Control mode• AUTO LIMIT lamp lights to indicate selection of automatic rudder limit function.16: Speed displays:• ROT in the Radius Control mode• Speed in the Heading Control mode• Speed warning indicator (LOG FAILURE or LOW)1-21. FURUNO Autopilot FAP-20001-31.3 Steering ModesThe FAP-2000 receives position, heading and speed data, compares them with the track section to be steered, and applies that information to calculate and command the necessary rudder angle.1.3.1 Hand steering modesThe following hand steering modes are available without the autopilot:• Steering wheel • Wing steering control • Override tillerWhile in a hand steering mode, the ECDIS indicates the rudder angle and the hand steering mode.1.3.2 Autopilot steering modesThe autopilot steering modes are selected from the autopilot control panel.Heading Control modeThe Heading Control steering mode can be used always because it does not require position data.• Mode selection: HEADING CONTROL• The HEADING CONTROL and HEADING lamps are lit. • Immediate course change when the tiller is used to set the heading. • Course change is defined as heading controlled by the set rudder angle limit. Radius Control modeThe Radius Control steering mode can be used always because it does not require position data.• Mode selection: RADIUS CONTROL• RADIUS CONTROL and HEADING lamps are lit. • Course change is radius controlled with the set radius.• If wind, current, etc. affect the ship, the ship will drift (inside or outside) from the planned turn. This is displayedon the radar screen.Program Heading Change modeThe Program Heading Change steering mode can be used always because it does not require position data.• Mode selection: PROGRAM HEADING CHANGE• PROGRAM HEADING CHANGE and HEADING lamps are lit.• The tiller is first used to set a new heading and radius, which are also displayed on the radar screen.• "START HEADING CHANGE" flashes if the newly set heading is different from the currently used heading. • Start course change by pushing the START HEADING CHANGE button. • After activation, control is returned to RADIUS CONTROL.• If wind, current, etc. affect the ship, the ship will drift away (inside or outside) from planned turn. This isdisplayed on the radar screen.1. FURUNO Autopilot FAP-20001-4Program Track modeThe Program Track steering mode requires the Kalman filter and a high-precision sensor such as DGPS:• Mode selection: PROGRAM TRACK• PROGRAM TRACK, TRACK and TURN lamps are lit.• The tiller is first used to set a new heading and radius, which are also displayed on the radar screen. • "START HEADING CHANGE" flashes if newly set heading is different from currently used set heading. • Start course change by pushing the START HEADING CHANGE button. • After activation, the mode becomes PROGRAM TRACK. • Course change is track controlled with the set radius.• If wind, current, etc. affect the ship, the system tries to prevent the ship from drifting away (inside or outside)from the planned designed turn. This is displayed on the radar screen. Alerts in the Program Track steering modeThe following alerts may appear in the Program Track steering mode.Alert "488 Track Control Stopped ": Internal failure - program track mode is cancelled.Alert "493 PrgTrack : Needs Filter ON ": The Kalman filter is not used with the program track mode. This alert is repeated every four minutes for the next 10 minutes. If the condition continues, the alert "496 ProgTrack : Stop - Sensor Fail " is generated and the steering mode is automatically changed to "Radius Control".Alert "494 PrgTrack : Needs Log sens.": The Kalman filter is used with the program track mode but withoutincluding an independent speed source (separate log sensor or two position sensors). This alert is repeated every four minutes for the next 10 minutes. If the condition continues, the alert "496 ProgTrack : Stop - Sensor Fail " is generated and the steering mode is automatically changed to "Radius Control".Alert "495 PrgTrack : Needs diff Pos.": The Kalman filter is used with the program track mode but without a high-precision sensor (for example, DGPS). This alert is repeated every four minutes for the next 10 minutes. If the condition continues, the alert "496 ProgTrack : Stop - Sensor Fail " is generated and the steering mode is automatically changed to "Radius Control".Alert "496 PrgTrack : Stop - Sensor Fail ": No gyro data or if conditions of alert 493, 494, 495 or 503 have been valid for the last 10 minutes.Alert "498 Use manual rudder control ": Generated every two minutes to alert the operator to control the rudder manually, when the FAP-2000 has lost the gyro data and thus cannot control the rudder.Alert "503 PrgTrack : Need higher Spd ": The Kalman filter is used with the program track mode but the speed is below the limit set for the track steering in the installation parameters. The alert is repeated every four minutes for the next 10 minutes. If the condition continues, the alert "496 ProgTrack : Stop - Sensor Fail " is generated and the steering mode is automatically changed to "Radius Control".Alert "504 PrgTrack : Use Radius ctrl.": Informs the operator to change the steering mode to "Radius Control". This alert is generated if there are not sufficient conditions to continue the program track (i.e. alert 493, 494, 495 or 503 has been valid two minutes). This alert is repeated every four minutes.Alert "509 PrgTrack : Need SOG/COG ref ": Appears when there is no Speed Over Ground (SOG) and Course Over Ground (COG) available from the position sensor(s) or bottom track from a dual-axis log. This alert isrepeated every four minutes for the next 10 minutes. If the condition continues, the alert "496 ProgTrack : Stop - Sensor Fail " is generated and the steering mode is automatically changed to "Radius Control".1. FURUNO Autopilot FAP-20001-5Summary of autopilot steering modesHEADING CONTROLRADIUS CONTROLPROGRAM HEADING CHANGEPROGRAM TRACKSet heading Set radius Radius control Design before execution Full curved EBL on radar screen Wind, current, etc. compensation Yes No No No No No Yes Yes Yes No Yes No Yes Yes Yes Yes Yes No Yes Yes Yes Yes Yes Yes Needs gyro Needs logNeeds high-precision position Needs direct SOG/COG sensorYes No No NoYes YesNo NoYes Yes No NoYes Yes Yes Yes1.3.3 Route steering modesIn route steering, you can use either the Goto WPT mode or the Goto Track mode. Route steering is available with a predefined monitored route and when your ship is located inside a channel of a monitored route.See the figure below for the differences between Goto WPT and Goto Track. As shown, the ship will always make way toward the waypoint in Goto WPT, and return to set course in Goto Track.WPTWPTWPTWPTWPT"Goto WPT"WPT“Goto Track"Note : If the off track error is more than 100 meters in the Goto Track mode, the system cannot increase the approach angle towards the center line of the route.1. FURUNO Autopilot FAP-20001-6Goto WPT mode • Mode selection: Goto WPT• Goto WPT and ROUTE lamps are lit.• The tiller can be used to set radius, but not course, which is set automatically. • Steering is course controlled with set radius.• If wind, current, etc. affect the ship between waypoints, the system tries to prevent the ship from drifting fromthe planned route. The system has three means to prevent drifting from the planned route, and they are most effective when used together. If cross-track error is used alone, your ship stabilizes typically in a constant off-track position. The means are:• Measured cross-track error from the centerline, which is always active. • Drift compensation available from route parameters • Gyro error compensation available from route parameters• If wind, current, etc. affect the ship during turns, the ship will drift (inside or outside) from the planned turn,which is displayed on the radar screen. This kind of turn is called an "assisted turn".• Can be used when the ship has an accurate position source available.• Normally, the Goto WPT mode uses the dynamic location of a waypoint. However, if alert 413 is active, a non-dynamic waypoint is used. The figure below shows how the location of a dynamic WPT is defined in the Goto WPT mode.used by "Goto Track"Goto Track mode • Mode selection Goto TRACK• Available when the ship has a high-precision position source available. • The GOTO TRACK button and ROUTE and TRACK lamps are lit.• The tiller can be used to set radius, but not heading, which is set automatically. • Steering is track-controlled with set radius.• If wind, current, etc. affect the ship between waypoints, the system tries to prevent the ship from drifting fromthe planned route. The system has three means to prevent drifting from the planned route, and they are most effective when used together. If cross-track error is used alone, your ship stabilizes typically in a constant off-track position. The means are:• Measured cross-track error from the centerline, which is always active. • Drift compensation is available from the route parameters. • Gyro error compensation is available from the route parameters. • The Goto Track mode uses non-dynamic waypoint.。
英语作文我最喜欢的汽车
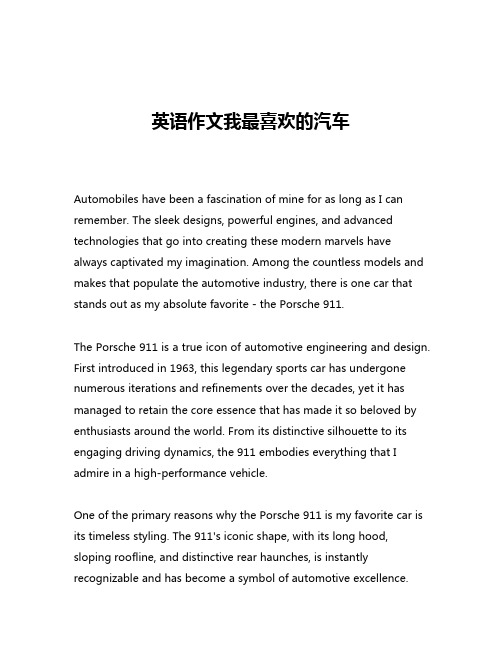
英语作文我最喜欢的汽车Automobiles have been a fascination of mine for as long as I can remember. The sleek designs, powerful engines, and advanced technologies that go into creating these modern marvels have always captivated my imagination. Among the countless models and makes that populate the automotive industry, there is one car that stands out as my absolute favorite - the Porsche 911.The Porsche 911 is a true icon of automotive engineering and design. First introduced in 1963, this legendary sports car has undergone numerous iterations and refinements over the decades, yet it has managed to retain the core essence that has made it so beloved by enthusiasts around the world. From its distinctive silhouette to its engaging driving dynamics, the 911 embodies everything that I admire in a high-performance vehicle.One of the primary reasons why the Porsche 911 is my favorite car is its timeless styling. The 911's iconic shape, with its long hood, sloping roofline, and distinctive rear haunches, is instantly recognizable and has become a symbol of automotive excellence.This design has evolved over the years, but it has always remained true to its original form, creating a sense of continuity and heritage that is unmatched by other sports car models.Beyond its striking appearance, the 911 also boasts an exceptional level of engineering and performance. At the heart of this car is a powerful, flat-six engine that delivers an exhilarating driving experience. The engine's unique configuration, with its horizontally-opposed cylinders, provides a low center of gravity and excellent balance, allowing the 911 to corner with precision and agility. The car's rear-engine layout also contributes to its exceptional handling characteristics, giving the driver a level of control and responsiveness that is truly remarkable.One of the things that I admire most about the Porsche 911 is its versatility. While it is undoubtedly a high-performance sports car, the 911 can also be a practical and comfortable daily driver. The car's interior is well-appointed and offers a surprising amount of space, making it suitable for both long road trips and daily commutes. Additionally, the 911's reputation for reliability and durability ensures that it can withstand the rigors of everyday use without sacrificing its performance capabilities.Another aspect of the Porsche 911 that I find particularly appealing is its rich heritage and racing pedigree. The 911 has a long and storiedhistory in motorsports, with numerous victories and championships to its name. From the iconic Porsche 917 that dominated the 24 Hours of Le Mans in the 1970s to the modern-day 911 RSR that competes in the World Endurance Championship, this car has proven its mettle on the world's most challenging racing circuits.This racing heritage has had a profound impact on the development of the 911, with the lessons learned on the track being directly applied to the road-going models. The result is a car that not only looks and performs like a thoroughbred sports car but also offers a level of refinement and sophistication that is rarely found in the world of high-performance automobiles.One of the most remarkable things about the Porsche 911 is its ability to evolve and adapt to changing times without losing its core identity. Over the decades, the 911 has undergone numerous technological and design advancements, yet it has always remained true to its original spirit. Whether it's the introduction of turbochargers, all-wheel-drive systems, or advanced electronic stability control, the 911 has seamlessly integrated these innovations while maintaining the engaging driving experience that has made it so beloved by enthusiasts.This ability to evolve while staying true to its roots is a testament to the vision and dedication of the Porsche engineering team. Theyhave consistently managed to strike the perfect balance between innovation and tradition, ensuring that the 911 remains a relevant and desirable sports car in an ever-changing automotive landscape.Beyond its technical merits, the Porsche 911 also holds a special place in my heart due to the emotional connection that I have with the car. There is something about the way the 911 makes you feel when you're behind the wheel that is truly unique. The sound of the engine, the precision of the steering, and the sheer exhilaration of the driving experience all come together to create a sense of pure joy and excitement that is unmatched by any other car I've driven.When I'm behind the wheel of a 911, I feel a deep sense of connection with the car and the rich history that it represents. It's as if I'm tapping into a lineage of automotive excellence that stretches back decades, and I'm honored to be a part of that tradition. The 911 is not just a car to me; it's a symbol of passion, performance, and a relentless pursuit of perfection.In conclusion, the Porsche 911 is my absolute favorite car for a multitude of reasons. From its timeless design and exceptional engineering to its rich heritage and emotional appeal, this iconic sports car has captured my heart and imagination in a way that no other automobile has. Whether I'm carving through winding mountain roads or simply admiring its stunning silhouette, the 911never fails to fill me with a sense of awe and wonder. It is a true masterpiece of automotive artistry, and I feel privileged to be able to call it my favorite car.。
汽车操控英语
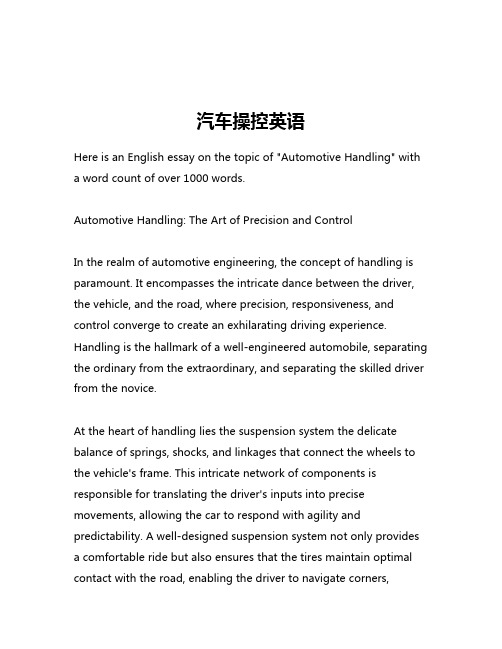
汽车操控英语Here is an English essay on the topic of "Automotive Handling" with a word count of over 1000 words.Automotive Handling: The Art of Precision and ControlIn the realm of automotive engineering, the concept of handling is paramount. It encompasses the intricate dance between the driver, the vehicle, and the road, where precision, responsiveness, and control converge to create an exhilarating driving experience. Handling is the hallmark of a well-engineered automobile, separating the ordinary from the extraordinary, and separating the skilled driver from the novice.At the heart of handling lies the suspension system the delicate balance of springs, shocks, and linkages that connect the wheels to the vehicle's frame. This intricate network of components is responsible for translating the driver's inputs into precise movements, allowing the car to respond with agility and predictability. A well-designed suspension system not only provides a comfortable ride but also ensures that the tires maintain optimal contact with the road, enabling the driver to navigate corners,straightaways, and unexpected obstacles with confidence.Equally crucial to handling is the steering system the mechanism that translates the driver's inputs at the steering wheel into directed wheel movements. The steering system must be tuned with meticulous care, striking a balance between responsiveness and stability. A heavy, sluggish steering feel can rob the driver of the connection to the road, while an overly sensitive setup can make the vehicle feel twitchy and unpredictable. The ideal steering system strikes a harmonious balance, providing the driver with a direct, linear feel that instills a sense of control and confidence.Braking is another essential element of handling. A vehicle's braking system must be capable of rapidly and consistently bringing the car to a halt, even in emergency situations. The interplay between the brake pads, calipers, and rotors is crucial, as is the overall brake system's ability to resist fade and maintain consistent pedal feel. Poorly designed brakes can compromise a vehicle's handling, leading to longer stopping distances, unpredictable behavior, and a loss of driver confidence.Beyond the mechanical components, the tires play a crucial role in handling. The tire's compound, tread pattern, and construction all contribute to its grip, responsiveness, and overall handling characteristics. A set of high-performance tires can transform aseemingly mundane vehicle into a responsive, agile machine, while the wrong tire choice can undermine even the most sophisticated suspension and steering systems.The driver's skill and technique are also integral to the handling equation. A skilled driver can extract the maximum performance from a vehicle, navigating corners with precision, managing weight transfer, and anticipating the car's behavior. Conversely, an unskilled driver may struggle to control a vehicle, leading to unpredictable and potentially dangerous situations.Ultimately, the art of automotive handling is a delicate balance of engineering, design, and human interaction. Automakers invest countless hours in the development and refinement of their vehicles' handling characteristics, meticulously tuning every component to achieve the perfect blend of responsiveness, stability, and control. For the driver, mastering the art of handling is a lifelong pursuit, a journey of understanding the vehicle, the road, and the dynamic interplay between the two.In the realm of high-performance driving, handling is the ultimate measure of a vehicle's capabilities. A car that excels in handling can navigate the most challenging roads with grace and confidence, inspiring the driver to push the limits of their own skills. Conversely, a vehicle with poor handling characteristics can be a source offrustration, limiting the driver's ability to explore the full potential of the machine.As the automotive industry continues to evolve, the importance of handling will only grow. Advancements in technology, such as active suspension systems, electronic stability control, and advanced tire compounds, are constantly pushing the boundaries of what's possible in terms of vehicle handling. The future of driving will likely be defined by the seamless integration of these technologies, creating automobiles that respond to the driver's inputs with unparalleled precision and control.In conclusion, the art of automotive handling is a multifaceted discipline that encompasses the interplay of engineering, design, and human interaction. It is a pursuit that demands the utmost attention to detail, a deep understanding of the vehicle's dynamics, and a keen sense of the driver's needs and preferences. As we continue to push the boundaries of what's possible in the world of automotive engineering, the importance of handling will only grow, ensuring that the driving experience remains a source of joy, excitement, and personal expression for generations to come.。
跑车模型的作文英语
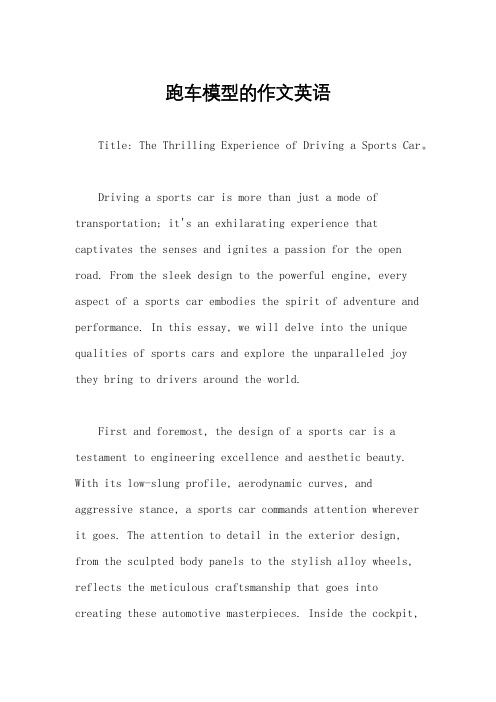
跑车模型的作文英语Title: The Thrilling Experience of Driving a Sports Car。
Driving a sports car is more than just a mode of transportation; it's an exhilarating experience that captivates the senses and ignites a passion for the open road. From the sleek design to the powerful engine, every aspect of a sports car embodies the spirit of adventure and performance. In this essay, we will delve into the unique qualities of sports cars and explore the unparalleled joy they bring to drivers around the world.First and foremost, the design of a sports car is a testament to engineering excellence and aesthetic beauty. With its low-slung profile, aerodynamic curves, and aggressive stance, a sports car commands attention wherever it goes. The attention to detail in the exterior design, from the sculpted body panels to the stylish alloy wheels, reflects the meticulous craftsmanship that goes intocreating these automotive masterpieces. Inside the cockpit,the driver is enveloped in luxury and comfort, surrounded by premium materials and cutting-edge technology. Every element of the interior is designed to enhance the driving experience, from the ergonomically designed seats to the intuitive infotainment system.However, it's under the hood where the true magic of a sports car comes to life. The heart of a sports car is its engine, a finely tuned marvel of engineering capable of delivering breathtaking performance. Whether it's a roaring V8 or a turbocharged inline-six, the sound of a sports car engine is music to the ears of enthusiasts. The acceleration is nothing short of exhilarating, pushing you back into your seat as you unleash the full power of the car. With each press of the throttle, you feel a surge of adrenaline coursing through your veins, propelling you forward with unbridled speed and agility.But it's not just about straight-line speed; sports cars are designed to excel in the corners as well. With precision steering and responsive handling, they hug the road like a second skin, allowing you to carve throughtwists and turns with confidence and finesse. The suspension is tuned for maximum grip and stability, keeping the car planted even under the most challenging driving conditions. Whether you're attacking a hairpin bend on a mountain pass or navigating a tight chicane on a race track, a sports car responds to your every command with unwavering precision.Of course, driving a sports car isn't just about performance; it's also about the sense of freedom and liberation it provides. There's something uniquelyliberating about hitting the open road in a powerful machine, the wind in your hair and the sun on your face. With the top down and the engine singing, you feel a senseof connection to the road and the world around you that is truly exhilarating. Whether you're cruising along a scenic coastline or blasting down a deserted highway, the experience of driving a sports car is unlike anything else.In conclusion, driving a sports car is an experiencethat transcends mere transportation. From the sleek designto the powerful engine, every aspect of a sports car isengineered to provide an unparalleled driving experience. Whether you're drawn to the adrenaline-pumping acceleration or the nimble handling, one thing is for certain: onceyou've experienced the thrill of driving a sports car,you'll never look at driving the same way again.。
横摆角速度英语

横摆角速度英语Yo, I'm all about that roll. When it comes to yaw rate,it's all about the speed of rotation around the vertical axis. It's crucial for maneuverability in vehicles, especially when you're looking to turn tight corners or make a sharp pivot.Understanding yaw rate is key to mastering control. It's not just about how fast you can turn, but how stable you can be while doing it. This is where the engineering and designof a vehicle come into play, ensuring that the yaw rate is optimized for performance and safety.In the world of aerodynamics, yaw rate is a game-changer. It affects the lift and drag forces acting on an aircraft, impacting its overall performance. Pilots and engineers work hand in hand to manage yaw rate to ensure a smooth andefficient flight.When it comes to racing, yaw rate is a critical factor. Drivers need to know how their car will respond to steering inputs, and yaw rate tells them just that. It's aboutprecision and control, making sure that every turn isexecuted with perfect timing.In the automotive industry, yaw rate sensors are a must. They provide real-time data on how the vehicle is rotating, which is vital for stability control systems. These systems use yaw rate information to adjust the vehicle's traction andbraking, ensuring a safe and stable ride.For those who love to push the limits, understanding and optimizing yaw rate can lead to thrilling experiences. Whether it's on the racetrack or just out for a spirited drive, mastering yaw rate can make all the difference between a good ride and a great one.In conclusion, yaw rate is an essential concept in the realm of motion and control. It's not just about turning;it's about how you turn and how well you can maintain control while doing so. So, keep your eye on the yaw rate, and you'll be turning heads in no time.。
轮胎英语1800字
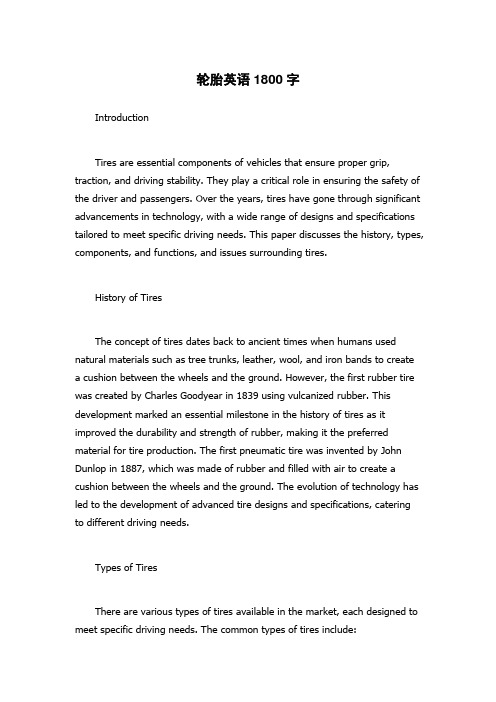
轮胎英语1800字IntroductionTires are essential components of vehicles that ensure proper grip, traction, and driving stability. They play a critical role in ensuring the safety of the driver and passengers. Over the years, tires have gone through significant advancements in technology, with a wide range of designs and specifications tailored to meet specific driving needs. This paper discusses the history, types, components, and functions, and issues surrounding tires.History of TiresThe concept of tires dates back to ancient times when humans used natural materials such as tree trunks, leather, wool, and iron bands to create a cushion between the wheels and the ground. However, the first rubber tire was created by Charles Goodyear in 1839 using vulcanized rubber. This development marked an essential milestone in the history of tires as it improved the durability and strength of rubber, making it the preferred material for tire production. The first pneumatic tire was invented by John Dunlop in 1887, which was made of rubber and filled with air to create a cushion between the wheels and the ground. The evolution of technology has led to the development of advanced tire designs and specifications, cateringto different driving needs.Types of TiresThere are various types of tires available in the market, each designed to meet specific driving needs. The common types of tires include:1. All-season tires - designed to operate in different weather conditions, including snow, rain, and dry conditions.2. Winter tires - optimized for use in freezing temperatures and heavy snow conditions, providing superior grip and traction control.3. Summer tires - designed for use in hot temperatures, providing improved steering and handling performance.4. Performance tires - designed for high-speed driving, improving grip and traction on racing tracks.5. Off-road tires - optimized for use in rough terrains, providing superior traction control and durability.Components of TiresTires are made up of several components, each contributing to their overall performance and durability. The components include:1. Tread - the part that comes into direct contact with the ground, providing traction and grip.2. Sidewall - the outer layer that protects the tire from damage and wear.3. Bead - the inner rubber layer that ensures the tire stays firm on the rim.4. Belts - the layer that reinforces the tire structure, providing additional strength and support.Functions of TiresTires play critical roles in ensuring the safety and performance of vehicles. The primary functions of tires include:1. Providing traction - tires provide grip and traction on the road surface, allowing the vehicle to accelerate, decelerate and turn effectively.2. Supporting the vehicle weight - tires are responsible for handling the weight of the vehicle, providing stability and balance.3. Absorbing shock - tires absorb impacts from road obstacles such as potholes and bumps, providing a smooth ride while protecting the vehicle’s suspension system.4. Steering - tires contribute to the vehicle’s steering performance, allowing the driver to turn and manoeuvre the vehicle effectively.Issues Surrounding TiresDespite the advancements in tire technology, several issues surround their safety and performance. The common issues include:1. Wear and tear - tires have a limited lifespan, and with constant use, their treads wear out, reducing their efficiency and safety.2. Punctures - tires are susceptible to punctures from sharp objects such as nails and stones, which may cause a blowout if not addressed.3. Underinflation - underinflated tires reduce fuel efficiency, reduce braking performance and increase the risk of a blowout.4. Over-inflation - over-inflated tires increase the risk of a blowout, reduce ride quality, and affect steering performance.ConclusionTires are essential components of vehicles that play critical roles in ensuring safety, performance, and driving experience. With the advancements in technology, there are various tire designs and specifications tailored to meet specific driving needs. It is essential to understand the types, components, functions, and issues surrounding tires to make informed decisions on tire selection, maintenance, and safety.。
汽车悬架英文
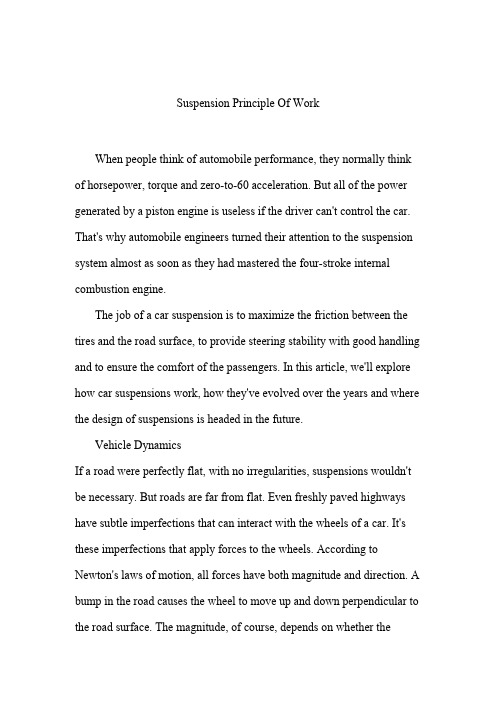
Suspension Principle Of WorkWhen people think of automobile performance, they normally think of horsepower, torque and zero-to-60 acceleration. But all of the power generated by a piston engine is useless if the driver can't control the car. That's why automobile engineers turned their attention to the suspension system almost as soon as they had mastered the four-stroke internal combustion engine.The job of a car suspension is to maximize the friction between the tires and the road surface, to provide steering stability with good handling and to ensure the comfort of the passengers. In this article, we'll explore how car suspensions work, how they've evolved over the years and where the design of suspensions is headed in the future.Vehicle DynamicsIf a road were perfectly flat, with no irregularities, suspensions wouldn't be necessary. But roads are far from flat. Even freshly paved highways have subtle imperfections that can interact with the wheels of a car. It's these imperfections that apply forces to the wheels. According to Newton's laws of motion, all forces have both magnitude and direction. A bump in the road causes the wheel to move up and down perpendicular to the road surface. The magnitude, of course, depends on whether thewheel is striking a giant bump or a tiny speck. Either way, the car wheel experiences a vertical acceleration as it passes over an imperfection.Without an intervening structure, all of wheel's vertical energy is transferred to the frame, which moves in the same direction. In such a situation, the wheels can lose contact with the road completely. Then, under the downward force of gravity, the wheels can slam back into the road surface. What you need is a system that will absorb the energy of the vertically accelerated wheel, allowing the frame and body to ride undisturbed while the wheels follow bumps in the road.The study of the forces at work on a moving car is called vehicle dynamics, and you need to understand some of these concepts in order to appreciate why a suspension is necessary in the first place. Most automobile engineers consider the dynamics of a moving car from two perspectives:Ride - a car's ability to smooth out a bumpy roadHandling - a car's ability to safely accelerate, brake and cornerThese two characteristics can be further described in three important principles - road isolation, road holding and cornering. The table below describes these principles and how engineers attempt to solve the challenges unique to each.A car's suspension, with its various components, provides all of the solutions described.Let's look at the parts of a typical suspension, working from the bigger picture of the chassis down to the individual components that make up the suspension proper.The ChassisThese systems include:The frame - structural, load-carrying component that supports the car's engine and body, which are in turn supported by the suspension The suspension system - setup that supports weight, absorbs and dampens shock and helps maintain tire contactThe steering system - mechanism that enables the driver to guide and direct the vehicleThe tires and wheels - components that make vehicle motion possible by way of grip and/or friction with the road So the suspension is just one of the major systems in any vehicle.SpringsToday's springing systems are based on one of four basic designs: Coil springs - This is the most common type of spring and is, in essence, a heavy-duty torsion bar coiled around an axis. Coil springs compress and expand to absorb the motion of the wheels.Leaf springs - This type of spring consists of several layers of metal (called "leaves") bound together to act as a single unit. Leaf springs were first used on horse-drawn carriages and were found on most American automobiles until 1985. They are still used today on most trucks and heavy-duty vehicles.Torsion bars - Torsion bars use the twisting properties of a steel bar to provide coil-spring-like performance. This is how they work: One end of a bar is anchored to the vehicle frame. The other end is attached to a wishbone, which acts like a lever that moves perpendicular to the torsion bar. When the wheel hits a bump, vertical motion is transferred to the wishbone and then, through the levering action, to the torsion bar. The torsion bar then twists along its axis to provide the spring force. European carmakers used this system extensively, as did Packard and Chrysler in the United States, through the 1950s and 1960s.Air springs - Air springs, which consist of a cylindrical chamber of air positioned between the wheel and the car's body, use the compressive qualities of air to absorb wheel vibrations. The concept is actually more than a century old and could be found on horse-drawn buggies. Air springs from this era were made from air-filled, leather diaphragms, much like a bellows; they were replaced with molded-rubber air springs in the 1930s.Shock AbsorbersUnless a dampening structure is present, a car spring will extend and release the energy it absorbs from a bump at an uncontrolled rate. The spring will continue to bounce at its natural frequency until all of the energy originally put into it is used up. A suspension built on springs alone would make for an extremely bouncy ride and, depending on the terrain, an uncontrollable car.Enter the shock absorber, or snubber, a device that controls unwanted spring motion through a process known as dampening. Shock absorbers slow down and reduce the magnitude of vibratory motions by turning the kinetic energy of suspension movement into heat energy that can be dissipated through hydraulic fluid. To understand how this works, it's best to look inside a shock absorber to see its structure and function.A shock absorber is basically an oil pump placed between the frame of the car and the wheels. The upper mount of the shock connects to theframe (i.e., the sprung weight), while the lower mount connects to the axle, near the wheel (i.e., the unsprung weight). In a twin-tube design, one of the most common types of shock absorbers, the upper mount is connected to a piston rod, which in turn is connected to a piston, which in turn sits in a tube filled with hydraulic fluid. The inner tube is known as the pressure tube, and the outer tube is known as the reserve tube. The reserve tube stores excess hydraulic fluid.When the car wheel encounters a bump in the road and causes the spring to coil and uncoil, the energy of the spring is transferred to the shock absorber through the upper mount, down through the piston rod and into the piston. Orifices perforate the piston and allow fluid to leak through as the piston moves up and down in the pressure tube. Because the orifices are relatively tiny, only a small amount of fluid, under great pressure, passes through. This slows down the piston, which in turn slows down the spring.Shock absorbers work in two cycles -- the compression cycle and the extension cycle. The compression cycle occurs as the piston moves downward, compressing the hydraulic fluid in the chamber below the piston. The extension cycle occurs as the piston moves toward the top of the pressure tube, compressing the fluid in the chamber above the piston.A typical car or light truck will have more resistance during its extension cycle than its compression cycle. With that in mind, the compressioncycle controls the motion of the vehicle's unsprung weight, while extension controls the heavier, sprung weight.All modern shock absorbers are velocity-sensitive -- the faster the suspension moves, the more resistance the shock absorber provides. This enables shocks to adjust to road conditions and to control all of the unwanted motions that can occur in a moving vehicle, including bounce, sway, brake dive and acceleration squat.Suspension Types: FrontSo far, our discussions have focused on how springs and dampers function on any given wheel. But the four wheels of a car work together in two independent systems -- the two wheels connected by the front axle and the two wheels connected by the rear axle. That means that a car can and usually does have a different type of suspension on the front and back. Much is determined by whether a rigid axle binds the wheels or if the wheels are permitted to move independently. The former arrangement is known as a dependent system, while the latter arrangement is known as an independent system. In the following sections, we'll look at some of the common types of front and back suspensions typically used on mainstream cars.Front Suspension - Dependent SystemsDependent front suspensions have a rigid front axle that connects thefront wheels. Basically, this looks like a solid bar under the front of the car, kept in place by leaf springs and shock absorbers. Common on trucks, dependent front suspensions haven't been used in mainstream cars for years.Front Suspension - Independent SystemsIn this setup, the front wheels are allowed to move independently. The MacPherson strut, developed by Earle S. MacPherson of General Motors in 1947, is the most widely used front suspension system, especially in cars of European origin.The MacPherson strut combines a shock absorber and a coil spring into a single unit. This provides a more compact and lighter suspension system that can be used for front-wheel drive vehicles.The double-wishbone suspension, also known as an A-arm suspension, is another common type of front independent suspension.Rear Suspension - Dependent SystemsIf a solid axle connects the rear wheels of a car, then the suspension is usually quite simple -- based either on a leaf spring or a coil spring. In the former design, the leaf springs clamp directly to the drive axle. The ends of the leaf springs attach directly to the frame, and the shock absorber is attached at the clamp that holds the spring to the axle. Formany years, American car manufacturers preferred this design because of its simplicity.Rear Suspension - Independent SuspensionsIf both the front and back suspensions are independent, then all of the wheels are mounted and sprung individually, resulting in what car advertisements tout as "four-wheel independent suspension." Any suspension that can be used on the front of the car can be used on the rear, and versions of the front independent systems described in the previous section can be found on the rear axles. Of course, in the rear of the car, the steering rack -- the assembly that includes the pinion gear wheel and enables the wheels to turn from side to side -- is absent. This means that rear independent suspensions can be simplified versions of front ones, although the basic principles remain the same.The Future of Car SuspensionsWhile there have been enhancements and improvements to both springs and shock absorbers, the basic design of car suspensions has not undergone a significant evolution over the years. But all of that's about to change with the introduction of a brand-new suspension design conceived by Bose -- the same Bose known for its innovations in acoustic technologies. Some experts are going so far as to say that the Bose suspension is the biggest advance in automobile suspensions since the introduction of an all-independent design.How does it work? The Bose system uses a linear electromagnetic motor (LEM) at each wheel in lieu of a conventional shock-and-spring setup. Amplifiers provide electricity to the motors in such a way that their power is regenerated with each compression of the system. The main benefit of the motors is that they are not limited by the inertia inherent in conventional fluid-based dampers. As a result, an LEM can extend and compress at a much greater speed, virtually eliminating all vibrations in the passenger cabin. The wheel's motion can be so finely controlled that the body of the car remains level regardless of what's happening at the wheel. The LEM can also counteract the body motion of the car while accelerating, braking and cornering, giving the driver a greater sense of control.。
- 1、下载文档前请自行甄别文档内容的完整性,平台不提供额外的编辑、内容补充、找答案等附加服务。
- 2、"仅部分预览"的文档,不可在线预览部分如存在完整性等问题,可反馈申请退款(可完整预览的文档不适用该条件!)。
- 3、如文档侵犯您的权益,请联系客服反馈,我们会尽快为您处理(人工客服工作时间:9:00-18:30)。
THE DESIGN OF A PERFORMANCE STEERING SYSTEM FOR COMPONENT-BASED GRID APPLICATIONS K.Mayes,G.D.Riley,R.W.Ford,M.Luj´a n,T.L.FreemanCentre for Novel Computing,University of ManchesterManchester M139PLken@griley@rupert@mlujan@lfreeman@C.AddisonComputing Services DepartmentUniversity of LiverpoolLiverpool L693BXcaddison@Abstract A major method of constructing applications to run on a computational Grid is toassemble them from components-separately deployable units of computation ofwell-defined functionality.Performance steering is an adaptive process involv-ing run-time adjustment of factors affecting the performance of an application.This paper presents a design for a system capable of steering,towards a mini-mum run-time,the performance of a component-based application executing ina distributed fashion on a computational Grid.The proposed performance steer-ing system controls the performance of single applications,and the basic designseeks to separate application-level and component-level concerns.The existenceof a middleware resource scheduler external to the performance steering systemis assumed,and potential problems are discussed.A possible model of opera-tion is given in terms of application and component execution phases.The needfor performance prediction capability,and for repositories of application-specificand component-specific performance information,is discussed.An initial im-plementation is briefly described.Keywords:performance steering,component-based application,Grids,Globus.21.IntroductionThe aim of this paper is to present a design for a system capable of controlling, or steering,the performance of a component-based application.A component is a unit of computation which has a well-defined functionality, and which can be composed with other components to create an application. Since a component can be individually deployed on to a hardware platform, component-based applications are,in the general case,distributed applications. Component-based applications are good candidates for deployment on to com-putational Grids,and several component frameworks exist to support the inter-action of components e.g.[9].It is in this context that performance steering of components and applications will be considered.Two kinds of computational steering have been identified:application steer-ing and performance steering[23].Application steering“lets researchers in-vestigate phenomena during the execution of their application...".On the other hand,performance steering“seeks to improve an application’s performance by changing application and resource parameters during runtime".Whereas appli-cation steering tends to be interactive,performance steering can be quantified and automated,allowing a long-running application to adapt to a changing ex-ecution environment.It would be equally valid to apply the term“performance control"to such an autonomous adaptive system.The term“performance steering"is defined here as being the adaptive process of adjusting,at run-time,factors affecting the performance of an application so that the application execution time is minimised.The performance steering system discussed here is being developed within the RealityGrid project[16].The aim of this project is to allow scientists to explore component-based simulations of physical phenomena.However,the project includes an investigation into autonomous performance steering of the simulations.This paper discusses possible execution environments for,and the nature of,component-based Grid applications,in order to motivate the performance steering design.The entities incorporated in the design are also discussed. Possible interactions with general Grid framework software are presented.An initial implementation of the basic design is briefly described,and issues to be investigated by incremental implementation are given.2.Execution environment of component-basedapplicationsIn order to design a performance steering system for a component-based application,it is necessary to examine the nature of such computations,and the features of the likely execution environment.Design of a Performance Steering System 3A schematic view of a simple component-based application executing on a computational Grid is shown in Figure 1.A computational Grid is a network of heterogeneous machines which can inter-operate to execute applications.The essence of a computational Grid is this inter-operation,supported by so-called grid middleware –a suite of more-or-less integrated software which interacts to provide security,resource management and information services.Although it is possible to implement component-based applications directly on this Grid middleware layer,it is also possible to have a further middleware layer to support components specifically.Such component frameworks allow greater flexibility and generality in the interactions between components.data generate display datacomponent 3component 1RESOURCESCOMPUTATIONAL GRID data process component 2Figure 1.An simple example of a component-based application.Central to such middleware layers is some resource scheduler ,responsi-ble for allocating Grid resources to applications submitted for execution.The GrADS project presents a Grid application execution framework which in-cludes a “scheduler/resource negotiator"[12].This scheduler is “responsible for choosing Grid resources appropriate for a particular problem run based on that run’s characteristics...".Similarly component frameworks,such as that being produced by the ICENI project,will include a scheduler which “can schedule according to the available resources and requirements"[9].Such Grid resource schedulers are referred to here as external resource sched-ulers because they are external to the performance steering system.An application,consisting of multiple components to be executed on a com-putational Grid,requires that multiple resources are allocated to it simulta-neously [5].There are two factors associated with resource scheduling for applications consisting of components which are of relevance here.The first is the allocation of a set of resources to the application as a whole.The second is the distribution of the components of the application over these resources.Few assumptions can be made about the nature of this external resource scheduler.At its simplest it may be in the form of a Globus Resource Spec-ification Language script written by the application owner [11].Alterna-tively,the scheduler could be one of the more sophisticated resource sched-ulers/negotiators.In the latter case,there must be some specification of the resource requirements of the application when it is submitted to the external resource scheduler.However,whilst considering the sophistication of possible4Grid resource schedulers,it is perhaps worth noting that“Currently,the most common current grid scheduler is the user"[19].2.1Resource allocation within a steered applicationIdeally,it would be assumed that the allocation and possibly initial distribu-tion of resources is carried out by the external resource scheduler,and that the run-time re-distribution of resources to components is the responsibility of the performance steering system.That is,a steered application would have afixed set of resources allocated to it.The partitioning of those resources between components of the application is under control of the performance steering system.Re-assignment of these given resources would enable the steering system to adjust the performance of its components.However,there may be specific hardware requirements associated with particular components(for example,a visualisation component)which would have to be taken into account.3.Performance steering of component-based applicationsIt is reasonable to assume that performance-steered applications will be exe-cuting in the presence of other applications running on the same computational Grid.However,the performance steering system cannot influence these other applications.That is,a steered application is steered in isolation.From the point of view of the performance steering system and its single steered application, all other applications are invisible.It is assumed that interactions between the resource requirements of com-peting applications,whether steered or not,will be dealt with by the external resource scheduler.Having an application-oriented approach to control perfor-mance efficiency for a single application is not new.The AppLeS scheduler emphasised application-specific information in meeting performance criteria [2].Similarly,in the GrADS framework the performance of each application is managed by its own“application manager"[12].3.1Application consists only of componentsA steered application consists only of interacting components.That is,it is assumed that there is no execution of application code external to the compo-nents.There is nothing that,for example,actively coordinates the behaviour of the components.This means that the performance of the application is de-termined solely by its components and their interactions.These interactions occur via the“middleware"which joins together the components,including the performance steering system.The performance steering system will not steer the performance of the middleware.Design of a Performance Steering System5 3.2Component performanceAn application is essentially aflow of control and of data through a set of interacting components.In general a component itself may be complex, consisting of several execution phases,where each phase can have different performance characterists or different resource requirements.Additionally,it may be possible to have alternative implementations of the same component, each using a different algorithm or data structure.There is,in the general case,no restriction on the interactions between com-ponents.That is,the performance of some set of components may limit the performance of the whole application.Since the performance characteristics of a component may change over its execution,different components may,at different points,become rate-limiting.That is,the critical path through the application may change.In order that a component can be steered,the component must provide some mechanisms or effectors which can be used by the steering system to modify the behaviour of the component.3.2.1Execution phases.The execution of the application,and its con-stituent components,is viewed as progressing through a series of phases.Each phase may have different characteristics from other phases.The usefulness of considering execution phases is that they enable performance to be char-acterised and measured at well-defined intermediate stages during run-time. Similarly,there is the possibility,between phases,of re-distributing resources to components.4.Application performance QoS and steeringIn an environment like a computational Grid,where an application may be running as processes on a diverse set of platforms,there is a need for Quality of Service(QoS)aware resource-sharing which prevents greedy applications from consuming resources shared with other applications[13].The provision of a QoS-aware resource scheduler can be based on predicting the application behaviour or resource usage,with subsequent run-time monitoring to detect failure to achieve the expected performance or usage.The main point here is that,in the case where there does exist some QoS performance contract for a Grid application,it is assumed that the external resource scheduler will be responsible for meeting QoS-related performance targets.This means that the role of the performance steering system is simply to optimise application performance with available resources.The sole criterion to be used for this optimisation will be execution time.It may happen that during run-time a QoS-aware external resource scheduler alters the allocation of resources and/or the distribution of components over6those resources.That is,both external resource scheduler and performance steerer would be re-distributing resources between components.This situation would require some coupling between scheduler and steerer in order to avoid chaos.resoutceexternalGRID COMPUTATIONAL RESOURCEScomponent 1componentperf steerercomponent perf steerer perf steerer component APSCPS CPS CPScomponent 2component 3scheduler performance steererapplicationFigure 2.The structure of an application consisting of three interacting components.Steering is provided at two levels:at the application level and at the component level.The application-level steerer (APS)controls the entire application via the component-level steerers (CPS).The Grid middleware is assumed to provide some external resource scheduler which is responsible for allocating resources to the application.5.Design of a performance steering systemHaving introduced issues about the execution environment and application structure,it is possible to describe the basic design of a performance steering system.As was shown in Figure 1,the components are distributed across the resources of a computational Grid.The entity which creates this initial distribution is some middleware external resource scheduler.In the general case,each component may be executing on a different platform from the others.Thus,in order to achieve maximum performance of each component,there should be some component-local performance steerer which has access to component-specific performance-related information and effectors.However,there needs also to be some entity which monitors the progress of the application as a whole,and which can,for example,redistribute allocated resources to components which have become rate-limiting.Design of a Performance Steering System7 Thus the basic design of the performance steering system is to divide func-tionality into two levels:the application level and the component level.That is,there is an Application Performance Steerer(APS)which is responsible for steering or controlling the performance of the application as a whole.At the component-level,each component has an associated Component Performance Steerer(CPS)which is responsible for steering or controlling the performance of only that component(Figure2).The APS is responsible for application-wide activity,such as re-distributing resources to components in order to affect performance.Similarly,any neces-sary interactions between the external resource scheduler and the performance steering system should occur via the APS.On the other hand,it is also assumed that there are component-specific behaviours that can be handled by the CPS, such as changing component performance by changing the algorithm used by a component.It is implicit in the roles of APS and CPS,that the steering system should have access to some performance prediction capability and to some repository of performance information.A more comprehensive design,showing these facilities added to the basic design,is given in Figure3.Additional features shown here include a resource usage monitor and a component loader responsi-ble for starting,restoring or migrating the component.In more concrete terms, this loader might be some“container"as in Enterprise Java Beans or ICENI[6] [10],or be implemented by some Unix server.6.Basic roles of APS and CPSWithin the context of this design,the APS and CPS have distinct roles.Both APS and CPS roles require some performance prediction facility.6.1Role of the APSThe role of the APS can be simply expressed:To distribute available resources amongst components such that the pre-dicted performance of components gives a minimum predicted application execution time.That is,the APS is given a set of resources by the external resource scheduler. The APS must attempt to distribute these resources between the components in an optimal manner,so that the application execution time will be minimised. Finding this optimal distribution involves:1predicting the performance of each component for a given resource dis-tribution;8applicationperformance steererAPS componentloaderperformance steerercomponent CPS predictorpredictor external schedulerrresourceperformanceapplicationrepository componentperformancerepositoryresourcesusagemonitor Figure 3.Entities in the performance steering system design.For simplicity,only one com-ponent is shown.2predicting the performance of the application for each set of predicted component performances.Thus performance prediction activity is central to the APS role.Performance-related information will be available via some performance repository.6.2Role of a CPSThe role of a CPS is simply expressed:To utilise resources and component performance effectors so that the pre-dicted execution time of the steered component is minimised.The APS allocates a set of resources to each component.Each CPS then steers its component so as to achieve minimum execution time using those resources.A CPS is assumed to have access to a repository of performance-related in-formation for that component.In addition,the CPS may take into account resource-usage information available for the platform on which the component is executing.Design of a Performance Steering System9 7.Performance steerer model of operation7.1APS model of operationOne possible view of the operation of the APS can be given in terms of a cycle of activities,shown in Figure4.An assumption made about an application to be performance-steered is that its execution proceeds in phases(see section3.2.1), and that there are progress points between the phases.APS activity is assumed to occur at these progress points.This APS activity is largely a decision-making process which may result in a new resource distribution.This simplified model allows activities to be considered more cleanly.That is,in this model all the APS activity occurs with synchronised arrival of all inputs to the APS decision-making process,and synchronised re-distribution of resources to components. In practice this synchronisation constraint will be relaxed.The synchronisation methods used will be determined on the basis of costs and benefits of possible mechanisms.However,the basic APS activities should be unchanged and can be described within the framework of the simple model presented.resourcesFigure4.A model of how the application performance steerer operates in the context of an application consisting of three components(C1,C2and C3).Execution proceeds in phases sep-arated by progress points.At each progress point the application performance steerer determines the best distribution of resources between components for the next phase.Arrows show possible inputs to this process.At each progress point,the APS generates a resource distribution for the com-ponents.The APS allocates resources such that the predicted performances of components will minimise application execution time.This implies that,at progress points,each component must be able to be restructured-the com-ponent must be at some“safepoint"in its execution.The CPSs then steer the performance of components through the next phase.107.2CPS model of operationAs with the application,each component can also be viewed as consisting of phases divided by progress points (for example,the timesteps in which the component may execute).However,there may be many component progress points within a one phase of the application (Figure 5).In this model,a CPS can steer its component autonomously between appli-cation progress points.In Figure 5,the CPS can steer its component during application phase 1,and to a lesser extent in phases 3and 4.During phase 2,there are no component progress points,so the CPS has very little opportunity to adjust to any resource changes which may be imposed by the APS.The greater the number of component progress points in an application phase,the greater is the opportunity for the CPS to steer its component during that phase.Greater autonomy for the CPS means less global activity,and opportunities for greater efficiency.01234phase 1phase 2phase 3phase 4applicationcomponentsteerercomponentsteererexecution timeapplication progress pointscomputation progress pointsFigure 5.A model of the relationship between computation,computation performance steerer and application performance rmation and control are exchanged at progress points indicated by the dotted vertical lines.There can be any number of computation progress points in one application phase.Application phases may be of different durations.8.Performance steering support facilitiesThe design presented in Figure 3includes performance repository,perfor-mance predictor and resource usage monitor facilities.These facilities are discussed in the following three subsections.Design of a Performance Steering System11 8.1Performance information repositoryThe proposed system will steer application performance by run-time adjust-ment of performance-related factors.These adjustments will be carried out on the basis of their expected effect on performance.That is,the performance steering system must be capable of making some sort of performance predic-tion1.The performance steering system will thus require information about the ap-plication in order to support performance prediction for the entire application. Similarly,component-specific performance-related data should also be avail-able to the system.Performance steering is an adaptive,run-time,process,and so information must be available for different algorithms,phases of execution, and resource configurations.This performance-related data will need to be available at run-time from some“repository".This repository must allow the addition and retrieval of a variety of performance history data,at run-time.The Global Grid Forum Grid Database Access and Integration Services Working Group are developing standards for database services on the Grid [1].It may be that this work can be used in designing the performance data repository.However,performance-related information has unique characteris-tics:it is usually short-lived,is updated more often than it is queried,and is often stochastic[22].Performance-related information includes:1information about the application and components,such as complexity of available algorithms,expected data and controlflow between compo-nents,component-specific hardware requirements.2models to compute expected execution behaviour for different platform configurations.3dynamic data about the previous performance history of the application: previously measured work-flows through its components,relation be-tween performance and number of processors,and so on.That is,performance-related data will need to be maintained about the per-formance of the application as a whole,and about the performance of each component.More specifically,it is apparent that there must be a division of the repository between dynamic short-lived data,and static long-lived data.The static portion of the repository should be optimised for queries whereas the dynamic portion should be optimised for updates.As an efficiency concern, 1The performance steering system is similar to a QoS-aware external resource scheduler in that it must co-schedule available resources to executables on the basis of some set of performance expectations.12component information should be maintained local to the component.The pro-cessing of dynamic history data into static data can occur“off-line"between runs,or during run-time.The repositories will be closely associated with the performance predictors of both APS and CPS.In addition,the APS may needa copy of data about components.8.2Performance predictionBoth the APS and CPS must have access to performance prediction capabil-ities of some sort.There is a great range of performance prediction methodolo-gies.These can perhaps be most simply split into two categories: parameters modelHere an analytical performance model is derived which can be parame-terised by application and system characteristics.The model is then used to generate performance predictions for specific application and system parameter values.Where system resource-related values are not constant, either monitored values or a range of values can be used[2][20].The approach can be application-specific or based on simple models[21]. Note that in the history heuristics methodology,there is no explicit perfor-mance prediction as such.The performance prediction appears to be implicit in the decision procedures and heuristics.The two approaches can perhaps be characterised(somewhat simplistically)as:newSys&ApplParameters heuristicPredictor(historyPerfData) predictedPerfData modelPredictor(sys&ApplParameters)More generally,in an application which is composed,perhaps dynamically, of components(or,in the Open Grid Services Architecture(OGSA)[8],of services),a performance model for the whole application may not be availableDesign of a Performance Steering System13 or may be difficult to derive.On the other hand,the problem of forming and usefully applying heuristics on a complex component-based distributed application may be non-trivial.It is possible that data-mining of history data may provide a useful approach to this problem.8.3Hardware resource-usage dataIn order to monitor the performance behaviour of the application compo-nents,it is necessary to have access to hardware resource-usage data.For ex-ample,if a component is not fully utilising all its resources,the steering system could choose to re-assign those unused resources to some other component, or even notify the external resource scheduler to free them.This resource-usage information could form part of the performance history data added to the performance repository.There are general-purpose resource-usage monitoring and predicting services (e.g.[24])which may be running on a computational Grid.There is an issue as to whether the performance steering system could or should interact with such general-purpose services.It may be sufficient to instrument the CPS,running on the same platform as the component,with calls to low-level monitoring facilities such as those available in the PAPI library[3].On multi-user platforms,there is an issue of whether the performance steerer should take account of usage perturbations due to other applications running on the same platforms,or simply assume that the steered application has sole use of the resources.Indeed,there is a general and difficult problem facing the performance steerer:that of balancing the costs and benefits of re-distributing resources to components.9.Generality of the performance steering system9.1Related workA major aim of work on Grid and component framework middleware is to simplify the task of running applications on computational Grids,whilst at the same time maintaining performance QoS for individual applications.The ICENI project emphasises a component framework for generality and simplicity of use[9].Application performance is achieved by an application mapper which selects the“best"component implementations for the resources available, based component meta-data.The GrADS project seeks to provide simplicity by building a framework for both preparing and executing Grid programmes[12]. Each application has an application manager which monitors the performance of that application for QoS achievement.Failure to achieve QoS contract causes a rescheduling or redistribution of resources.Both ICENI and GrADS have resource schedulers which partition resources between applications–described。