Dark Matter Direct Searches and the Anomalous Magnetic Moment of Muon
暗物质成品

What is dark matter?
About 65 years ago, the first time that evidence of the existence of dark matter. At that time, Fulizizha Popovich found a large cluster of galaxies in the galaxy has a very high velocity, unless the quality of galaxy clusters is based on the number of calculations in which stars are more than 100 times the value, or cluster of galaxies can not bound lives of these galaxies. After decades of observation and analysis confirmed this. Although the nature of dark matter is still unknown, but by 80 years, accounting for about 20% of the energy density of dark matter to be widely accepted.
What is dark matter?
now we know that dark matter has become an important part of the universe. The total mass of dark matter is ordinary matter, 6.3 times the energy density in the universe, accounting for 1 / 4, but also important is that dark matter dominated the formation of cosmic structures. Now the nature of dark matter remains a mystery, but assuming it is a weak interaction of subatomic particles, then the resulting large-scale structure of the universe is consistent with the observations. Recently, however, the structure of galaxies and galaxy subanalysis shows that this assumption and the difference between observations, which at the same time provide a variety of possible dark matter theory was useless. Small-scale structure through the density, distribution, evolution and its environment studies can distinguish between these potential dark matter model for the nature of dark matter to bring a new dawn.
Planned Dark Matter searches with the MAGIC Telescope
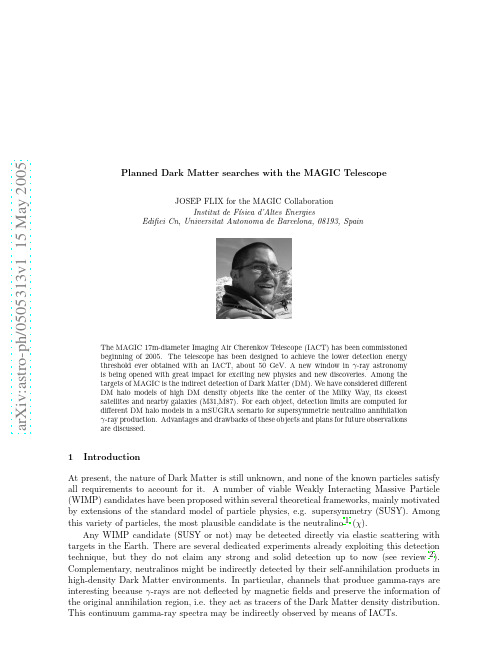
a r X i v :a s t r o -p h /0505313v 1 15 M a y 2005Planned Dark Matter searches with the MAGIC Telescope JOSEP FLIX for the MAGIC Collaboration Institut de F´ısica d’Altes Energies Edifici Cn,Universitat Autonoma de Barcelona,08193,Spain The MAGIC 17m-diameter Imaging Air Cherenkov Telescope (IACT)has been commissioned beginning of 2005.The telescope has been designed to achieve the lower detection energy threshold ever obtained with an IACT,about 50GeV.A new window in γ-ray astronomy is being opened with great impact for exciting new physics and new discoveries.Among the targets of MAGIC is the indirect detection of Dark Matter (DM).We have considered different DM halo models of high DM density objects like the center of the Milky Way,its closest satellites and nearby galaxies (M31,M87).For each object,detection limits are computed for different DM halo models in a mSUGRA scenario for supersymmetric neutralino annihilation γ-ray production.Advantages and drawbacks of these objects and plans for future observationsare discussed.1IntroductionAt present,the nature of Dark Matter is still unknown,and none of the known particles satisfy all requirements to account for it.A number of viable Weakly Interacting Massive Particle (WIMP)candidates have been proposed within several theoretical frameworks,mainly motivated by extensions of the standard model of particle physics,e.g.supersymmetry (SUSY).Among this variety of particles,the most plausible candidate is the neutralino 1(χ).Any WIMP candidate (SUSY or not)may be detected directly via elastic scattering with targets in the Earth.There are several dedicated experiments already exploiting this detection technique,but they do not claim any strong and solid detection up to now (see review 2).Complementary,neutralinos might be indirectly detected by their self-annihilation products in high-density Dark Matter environments.In particular,channels that produce gamma-rays are interesting because γ-rays are not deflected by magnetic fields and preserve the information of the original annihilation region,i.e.they act as tracers of the Dark Matter density distribution.This continuum gamma-ray spectra may be indirectly observed by means of IACTs.2The MAGIC ExperimentTo date,the Major Atmospheric Imaging Cerenkov telescope(MAGIC3)is the largest world-wide Imaging Air Cerenkov Telescope(IACT).Located on the Canary Island La Palma(2200m a.s.l),the telescope has a17m diameter high reflectivity tessellated parabolic mirror dish, mounted on a light weight carbonfiber frame.It is equipped with a high efficiency576-pixel photomultiplier camera,whose analogue signals are transported via opticalfibers to the trigger electronics and the300MHz FADC readout.Its physics program comprises,among other topics, pulsars,supernova remnants,active galactic nuclei,micro-quasars,gamma-ray bursts and Dark Matter.Several positive detections of already knownγ-ray emitters have been already accomplished during its initial phase(see contributions to these proceedings from N.Tonello[1ES1959+650], M.L´o pez[Crab Nebula]and D.Mazin[Mrk-421]).Coping with the hadronic background below 100GeV presents a new challenge,but these observations evidence that MAGIC analysis extends well below100GeV and,encouragingly enough,evenγ-rays with an estimated energy of50 GeV trigger the telescope.The analysis methods are presently being adapted to the low-energy domain,uncharted territory as yet.Meanwhile,the collaboration is involved in the construction of a second telescope(MAGIC-II,see A.Moralejo contribution to these proceedings).This will improve the angular and spectral resolution andflux sensitivity of the system.3Gamma-rays from neutralino annihilationsNeutralino annihilation can generate continuumγ-ray emission,via the processχχ→q¯q.The subsequent decay ofπ0-mesons created in the resulting quark jets produces a continuum ofγ-rays.The expected annihilationγ-rayflux above an energy E thresh arriving at Earth is given by:dNγ(Eγ>E thresh)2·σv3.1Galactic CenterThe presence of a Dark Matter halo in the Milky Way Galaxy is well established by stellar dynamics.In particular,stellar rotation curve data of the Milky Way can be adjusted byfitting with the use of universal DM profiles predicted by simulations.The most recent models include an effect that had been previously neglected and affects the DM density at the center of the Milky Way halo,namely the compression of the Dark Matter due to the infall of baryons to the innermost region5.As Dark Matter density is enhanced at the center the expected signal is boosted.This model is based on a large amount of observational data of our galaxy and is in good agreement with the brand new cosmological simulations for the response of Dark Matter halos to condensation of baryons6.Such a central spike in the center of the galaxy is under debate and depend on the Black Hole history during galaxy formation.There exist some evidences against a central cusp,but they must be taken with caution(see F.Ferrer in these proceedings).We consider an uncompressed NFW DM halo model7and the adiabatic contracted NFW profile5.3.2Draco dwarf spheroidal and nearby galaxiesThe Milky Way is surrounded by a number of small and faint companion galaxies.These dwarf satellites are by far the most Dark Matter dominated known objects,with Mass-to-Light ratios from30to300.Draco is the most DM dominated dwarf satellite.DM density profiles derived from Draco stars cannot differentiate between cusped or cored profiles in the innermost region, as data are not available at small radial distances.Moreover,observational data disfavors tidal disruption effects,which may affect dramatically the DM distribution in Draco.In order to compare with the Galactic Center we adopt the very recent cusped DM model which includes new available Draco data8.In addition,we adopted NFW models for the nearby galaxy M319and the Virgo Cluster10. These NFW profiles do not take into account any enhancement effect,like adiabatic contraction or presence of DM substructures.4SummaryFigure1shows exclusion limits for MAGIC in the mSUGRA plane Nγ(Eγ>E thresh) σv vs. mχfor the four most promising sources considered.The nominal energy threshold E thresh has been assumed to be conservatively100GeV and has been accordingly scaled with the zenith angle observation,as well as the telescope collection area.The expectedfluxes are rather low and depends strongly on the innermost density region of the DM halos considered.The detection of a DMγ-ray signal from the Galactic Center is possible(or achievable)in case of a very high density DM halo,like the one predicted by adiabatic contraction processes(expected improvements on the E thresh allows to test a significant portion of the SUSY parameter space).Theflux measured by the HESS(see L.Rolland in these proceedings)experiment is far above the theoretical expectations(it extends to the TeV regime), indicating that the observed gamma radiation is most probably not due to the annihilation of SUSY-neutralino Dark Matter particles.Nevertheless,other models like Kaluza-Klein Dark Matter are not ruled out.It is interesting to investigate and characterize the observed gamma radiation to constrain the nature of the emission.In addition,it is not excluded that a part of theflux is due to Dark Matter annihilation.Due to the large zenith angle for Galactic Center observations,MAGIC will have a large energy threshold but also a large collection area and good statistics at the highest energies.Galactic Center observations are foreseen from April to August200511.Figure1:Exclusion limits for the four most promising sources of Dark Matter annihilation radiation.The Galactic Center is expected to give the largestflux(lowest exclusion limits)amongst all sources.In the long term we consider Draco as a plausible candidate for Dark Matter inspired observa-tions.Conservative scenarios give lowfluxes which are not detectable by MAGIC in a reasonable observation time.However,there are several factors that might enhance the expectedflux from neutralino annihilations in Draco.Other Dark Matter particles,like Kaluza-Klein particles,may produce gamma-rays,maybe with a higherflux than those quoted here.Draco is the most DM dominated dwarf(M/L up to300)and an object where no otherγ-ray emission is expected. Low zenith angle observations will preserve the nominal(low)E thresh of the MAGIC telescope. Moreover,no known VHE emitters in the FOV provides no otherγ-ray sources in competition to the one predicted in the exposed DM scenario.4.1AcknowledgmentsI am grateful to the Conference Organizers for a very enjoyable week and Conference in La Thuille and to all the members of the MAGIC Dark Matter working group for fruitful discussions.References1.G.Jungman,M.Kamionkowski and K.Griest,Physics Reports,267,195-373(1996)2.J.Gascon,astro-ph/05042413.J.Cortina for the MAGIC Collaboration,Astrophys.Space Sci.297,245-255(2005)4.J.Navarro,C.Frenk and S.White,ApJ490,493(1997)5.F.Prada,A.Klypin,J.Flix et al.,Phys.Rev.Lett.93,241301(2004)6.O.Gnedin et al.,Astrophys.J.61616-26(2004)7.N.Fornengo et al.,Phys.Rev.70103529(2004)8.E.Lokas et al.,submitted to MNRAs(2005)9.N.W.Evans et al.,Phys.Rev.D6*******(2004)10.D.E.McLaughlin,ApJ512L9(1999)11.MAGIC Dark Matter Working Group,J.Flix,Phd.Thesis(2005)。
暗物质_中学教育-中考
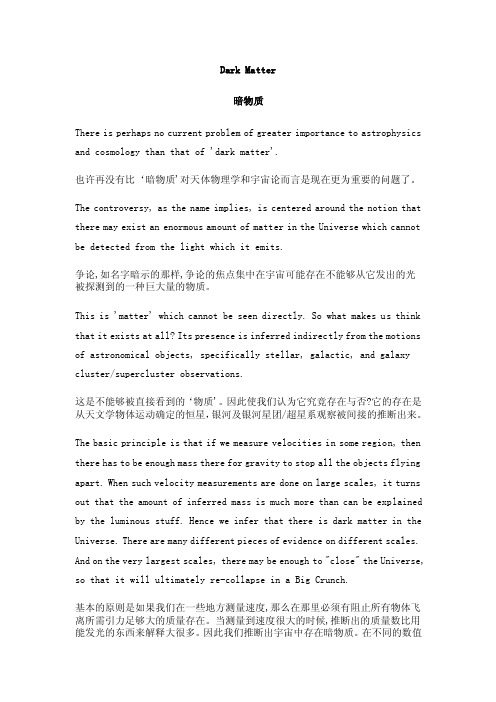
Dark Matter暗物质There is perhaps no current problem of greater importance to astrophysics and cosmology than that of 'dark matter'.也许再没有比‘暗物质'对天体物理学和宇宙论而言是现在更为重要的问题了。
The controversy, as the name implies, is centered around the notion that there may exist an enormous amount of matter in the Universe which cannot be detected from the light which it emits.争论,如名字暗示的那样,争论的焦点集中在宇宙可能存在不能够从它发出的光被探测到的一种巨大量的物质。
This is 'matter' which cannot be seen directly. So what makes us think that it exists at all? Its presence is inferred indirectly from the motions of astronomical objects, specifically stellar, galactic, and galaxy cluster/supercluster observations.这是不能够被直接看到的‘物质'。
因此使我们认为它究竟存在与否?它的存在是从天文学物体运动确定的恒星,银河及银河星团/超星系观察被间接的推断出来。
The basic principle is that if we measure velocities in some region, then there has to be enough mass there for gravity to stop all the objects flying apart. When such velocity measurements are done on large scales, it turns out that the amount of inferred mass is much more than can be explained by the luminous stuff. Hence we infer that there is dark matter in the Universe. There are many different pieces of evidence on different scales. And on the very largest scales, there may be enough to "close" the Universe, so that it will ultimately re-collapse in a Big Crunch.基本的原则是如果我们在一些地方测量速度,那么在那里必须有阻止所有物体飞离所需引力足够大的质量存在。
05-15英语一新题型整理版完美打印版

How does your reading proceed? Clearly, you try to comprehend, in the sense of identifying meanings for individual words and working out relationships between them, drawing on your implicit knowledge of English grammar.(41)________________. You begin to infer a context for the text, for instance, by making decisions about what kind of speech event is evolved. Who is making the utterance, to whom, when and where?The ways of reading indicated here are without doubt kinds of comprehension but they show comprehension to consist not just of passive assimilation but of active engagement in inference and problem-solving. You infer information you feel the writer has invited you to grasp by presenting you with specific evidence and clues. (42) ________________ Conceived in this way, comprehension will not follow exactly the same track for each reader. What is in question is not the retrieval of an absolute, fixed or ―true‖ meaning that can be read off and checked for accuracy, or some timeless relationship of the text to the world. (43) _____________Such background material inevitably reflects who we are. (44) _____________. This does not, however, make interpretation merely relative or even pointless. Precisely because readers from different historical periods, places and social experiences produce different but overlapping readings of the same words on the page---including for texts that engage with fundamental human concerns--- debates about texts can play an important role in social discussion of belief and values.How we read a given text also depends to some extent on our particular interest in reading it. (45) _____________. Such dimensions of reading suggest---as others introduced later in the book will also do--that we bring an implicit (often unacknowledged) agenda to any act of reading. It does not then necessarily follow that one kind of reading is fuller, more advanced or more worthwhile than another. Ideally, different minds of reading inform each other, and act as useful reference points for and counterbalances to one another. Together, they make up the reading component of your overall literacy, or relationship to your surrounding textual environment.[A] Are we studying that text and trying to respond in a way that fulfills the requirement of a given course? Reading it simply for pleasure? Skimming it for information? Ways of reading on a train or in bed are likely to differ considerably from reading in a seminar room.[B] Factors such as the place and period in which we are reading, our gender ethnicity, age and social class will encourage us towards certain interpretations but at the same time obscure or even close off others.[C] If you are unfamiliar with words or idioms, you guess at their meaning, using clues presented in the context. On the assumption that they will become relevant later, you make a mental note of discourse entities as well as possible links between them.[D]In effect, you try to reconstruct the likely meanings or effects that any given sentence, image or reference might have had: These might be the ones the author intended.[E] You make further inferences, for instance, about how the text may be significant to you, or about its validity—inferences that form the basis of a personal response for which the author will inevitably be far less responsible.[F] In plays, novels and narrative poems, characters speak as constructs created by the author, not necessarily as m outhpieces for the author’s own thoughts.[G]Rather, we ascribe meanings to texts on the basis of interaction between what we might call textual and contextual material: between kinds of organizations or patterning we perceive in a text’s formal structur es (so especially its language structures) and various kinds of background, social knowledge, belief and attitude that we bring to the text.[A] Some archaeological sites have always been easily observable—for example, the Parthenon in Athens, Greece; the pyramids of Giza in Egypt; and the megaliths of Stonehenge in southern England. But these sites are exceptions to the norm .Most archaeological sites have been located by means of careful searching, while many others have been discovered by accident. Olduvai Gorge, fell into its deep valley in 1911.Thousands of Aztec artifacts came to light during the digging of the Mexico City subway in the 1970s.[B] In another case, American archaeologists Rene million and George Cowgill spent years systematically mapping the entire city of Teotihuacan in the valley of Mexico near what is now Mexico City .at its peak around AD 600, this city was one of the largest human settlements in the word. The researchers mapped not only the city’s vast and ornate ceremo nial areas, but also hundreds of simpler apartment complexes where common people lived.[C] How do archaeologists know where to find what they are looking for when there is nothing visible on the surface of the ground? Typically, they survey and sample (make test excavations on) large areas of terrain to determine where excavation will yield useful information. Surveys and test samples have also become important for understanding the larger landscapes that contain archaeological sites.[D] Surveys can cover a single large settlement or entire landscapes. In one case, many researchers working around the ancient Maya city of Copán, Honduras, have located hundreds of small rural village and individual dwellings by using aerial photographs and by making surveys on foot. The resulting settlement maps show how the distribution and density of the rural population around the city changed dramatically between AD500 and 850, when Copán collapsed.[E] To find their sites, archaeologists today rely heavily on systematic survey methods and a variety of high-technology tools and techniques. Airborne technologies, such as different types of radar and photographic equipment carried by airplanes or spacecraft, allow archaeologists to learn about what lies beneath the ground without digging. Aerial surveys locate general areas of interest or larger buried features, such as ancient buildings or fields.[F] Most archaeological sites, however, are discovered by archaeologists who have set out to look for them. Such searches can take years. British archaeologist Howard Carter knew that the tomb of the Egyptian pharaoh Tutankhamen existed from information found in other sites. Carter sifted through rubble in the Valley of the King for seven years before he located the tomb in 1922. In the late 1800s British archaeologist Sir Arthur Eyan combed antique dealers’ stores in Athens, Greece. He was searching for thing engraved seals attributed to the ancient Mycenaean culture that dominated Greece from the 1400s to 1200s BC. Evas’s inter pretations of those engravings eventually led them to find the Minoan palace at Knossos on the island of Crete, in 1900.[G] Ground surveys allow archaeologists to pinpoint the places where digs will be successful. Most ground surveys involve a lot of walking, looking for surface clues such as small fragments of pottery. They often include a certain amounts of digging to test for buried materials at selected points across a landscape. Archaeologists also may locate buried remains by using such technologies as ground radar, magnetic-field recording, and metal detector. Archaeologists commonly use computers to map sites and the landscapes around sites. Two and three-dimensional maps are helpful tools in planning excavations, illustrating how sites look, and presenting the results of archaeological research.41 --- A --- 42. --- E ---43 --- 44 --- 45The social sciences are flourishing.As of 2005,there were almost half a million professional social scientists from all fields in the world, working both inside and outside academia. According to the World Social Science Report 2010,the number of social-science students worldwide has swollen by about 11% every year since 2000.Yet this enormous resource in not contributing enough to today’s globa l challenges including climate change, security,sustainable development and health.(41)______Humanity has the necessary agro-technological tools to eradicate hunger , from genetically engineered crops to arificial fertilizers . Here , too, the problems are social: the organization and distribution of food, wealth and prosperity.(42)____This is a shame—the community should be grasping the opportunity to raise its influence in the real world. To paraphrase the great social scientist Joseph Schumpeter:there is no radical innovation without creative destruction .Today ,the social sciences are largely focused on disciplinary problems and internal scholarly debates,rather than on topics with external impact.Analyses reveal that the number of papers including th e keywords ―environmental changed‖ or ―climate change‖ have increased rapidly since 2004,(43)____When social scientists do tackle practical issues ,their scope is often local:Belgium is interested mainly in the effects of poverty on Belgium for example .A nd whether the community’s work contributes much to an overall accumulation of knowledge is doubtful.The problem is not necessarily the amount of available funding (44)____this is an adequate amount so long as it is aimed in the right direction. Social sc ientists who complain about a lack of funding should not expect more in today’s economic climate.The trick is to direct these funds better.The European Union Framework funding programs have long had a category specifically targeted at social scientists.This year,it was proposed that system be changed:Horizon 2020,a new program to be enacted in 2014,would not have such a category ,This has resulted in protests from social scientists.But the intention is not to neglect social science ; rather ,the complete opposite.(45)____That should create more collaborative endeavors and help to develop projects aimed directly at solving global problems.[A] It could be that we are evolving two communities of social scientists:one that is discipline-oriented and publishing in highly specialized journals,and one that is problem-oriented and publishing elsewhere,such as policy briefs.[B] However,the numbers are still small:in 2010,about 1,600 of the 100,000 social-sciences papers published globally included one of these Keywords.[C] the idea is to force social to integrate their work with other categories, including health and demographic change food security, marine research and the bio-economy, clear, efficient energy; and inclusive, innovative and secure societies.[D] the solution is to change the mindset of the academic community, and what it considers to be its main goal. Global challenges and social innovation ought to receive much more attention from scientists, especially the young ones.[E] These issues all have root causes in human behavior . all require behavioral change and social innovations , as well as technological development . Stemming climate change , for example , is as much about changing consumption patterns and promoting tax acceptance as it is about developing clean energy.[F] Despite these factors , many social scientists seem reluctant to tackle such problems . And in Europe , some are up in arms over a proposal to drop a specific funding category for social-science research and to integrate it within cross-cutting topics of sustainable development .[G] During the late 1990s , national spending on social sciences and the humanities as a percentage of all research and development funds-including government, higher education, non-profit and corporate -varied from around 4% to 25%; inThink of those fleeting moments when you look out of an aeroplane window and realise that you are flying, higher than a bird. Now think of your laptop, thinner than a brown-paper envelope, or your cellphone in the palm of your hand. Take a moment or two to wonder at those marvels. You are the lucky inheritor of a dream come true.The second half of the 20th century saw a collection of geniuses, warriors, entrepreneurs and visionaries labour to create a fabulous machine that could function as a typewriter and printing press, studio and theatre, paintbrush and gallery, piano and radio, the mail as well as the mail carrier. (41)The networked computer is an amazing device, the first media machine that serves as the mode of production, means of distribution, site of reception, and place of praise and critique. The computer is the 21st century's culture machine.But for all the reasons there are to celebrate the computer, we must also tread with caution. (42)I call it a secret war for two reasons. First, most people do not realise that there are strong commercial agendas at work to keep them in passive consumption mode. Second, the majority of people who use networked computers to upload are not even aware of the significance of what they are doing.All animals download, but only a few upload. Beavers build dams and birds make nests. Yet for the most part, the animal kingdom moves through the world downloading. Humans are unique in their capacity to not only make tools but then turn around and use them to create superfluous material goods - paintings, sculpture and architecture - and superfluous experiences - music, literature, religion and philosophy. (43)For all the possibilities of our new culture machines, most people are still stuck in download mode. Even after the advent of widespread social media, a pyramid of production remains, with a small number of people uploading material, a slightly larger group commenting on or modifying that content, and a huge percentage remaining content to just consume.(44)Television is a one-way tap flowing into our homes. The hardest task that television asks of anyone is to turn the power off after he has turned it on.(45)What counts as meaningful uploading? My definition revolves around the concept of "stickiness" - creations and experiences to which others adhere.[A] Of course, it is precisely these superfluous things that define human culture and ultimately what it is to be human. Downloading and consuming culture requires great skills, but failing to move beyond downloading is to strip oneself of a defining constituent of humanity.[B] Applications like , which allow users to combine pictures, words and other media in creative ways and then share them, have the potential to add stickiness by amusing, entertaining and enlightening others.[C] Not only did they develop such a device but by the turn of the millennium they had also managed to embed it in a worldwide system accessed by billions of people every day.[D] This is because the networked computer has sparked a secret war between downloading and uploading - between passive consumption and active creation - whose outcome will shape our collective future in ways we can only begin to imagine.[E] The challenge the computer mounts to television thus bears little similarity to one format being replaced by another in the manner of record players being replaced by CD players.[F] One reason for the persistence of this pyramid of production is that for the past half-century, much of the world's media culture has been defined by a single medium - television - and television is defined by downloading.[G]The networked computer offers the first chance in 50 years to reverse the flow, to encourage thoughtful downloading[A] No disciplines have seized on professionalism with as much enthusiasm the humanities. You can, Mr. Menand points out, became a lawyer in three years and a medical doctor in four. But the regular time it takes to get a doctoral degree in the humanities is nine years. Not surprisingly, up to half of all doctoral students in English drop out before getting their degrees.[B] His concern is mainly with the humanities: Literature, languages, philosophy and so on. These are disciplines that are going out of sytle:22% of American college graduates now major in business compared with only 2% in history and 4% in English. However, many leading American universities want their undergraduates to have a grounding in the basic canon of ideas that every educated person should posses. But most find it difficult to agree on what a ―general education‖ should look like. At Harvard, Mr. Menand notes, ―the great books are read because they have been read‖, they form a sort of social glue.[C] Equally unsurprisingly, only about half end up with professorships for which they entered graduate school. There are simply too few posts. This is partly because universities continue to produce ever more PhDs. But fewer students want to study humanities subjects: English department awarded more bachelor’s degrees in 1970-71 than they did 20 years later. Fewer students requires fewer teachers. So, at the end of a decade of theses-writing, many humanities students leave the profession to du something for which they have not been trained.[D] One reason why it is hard to design and teach courses is that they can cut across the insistence by top American universities that liberal-arts educations and professional education should be kept separate, taught in different schools. Many students experience both varieties Although more than half of Harvard undergraduates end up in law, medicine or business, future doctors and lawyers must study a non-specialist liberal-art degree before embarking on a professional qualification.[E] Besides professionalizing the professions by this separation top American universities have professionalized the professor. The growth on public money for academic research has speeded the process: federal research grants rose fourfold between 1960 and 1990, but faculty teaching hours fell by half as research took its toll. Professionalism has turned the acquisition of a doctoral degree into a prerequisite for a successful academic career: as late as 1969 a third of American professors did not possess one. But the key idea behind professionalization, argues Mr. Menand, is that ―the knowledge and skills needed for a particular specialization are transmissible but not transferab le.‖ So disciplines acquire a monopoly not just over the production of knowledge, but also over the production of the producers of knowledge.[F] The key to reforming higher education, concludes Mr. Menand, is to alter the way in which ―the producers of k nowledge are produced.‖ Otherwise, academics will continue to think dangerously alike, increasingly detached from the societies which they study, investigate and criticize. ―Academic inquiry, at least in some fields, may need to become less exclusionary an d more holistic.‖ Yet quite how that happens, Mr. Menand dose not say.[G] The subtle and intelligent little book The marketplace of Ideas: Reform and Resistance in the American University should be read by every student thinking of applying to take a doctoral degree. They may then decide to go elsewhere. For something curious has been happening in American Universities, and Louis Menand, a professor of English at Harvard University, captured it skillfully.[A] The first and more important is the consumer's growing preference for eating out; the consumption of food and drink in places other than homes has risen from about 32 percent of total consumption in 1995 to 35 percent in 2000 and is expected to approach 38 percent by 2005. This development is boosting wholesale demand from the food service segment by 4 to 5 percent a year across Europe, compared with growth in retail demand of 1 to 2 percent. Meanwhile, as the recession is looming large, people are getting anxious. They tend to keep a tighter hold on their purse and consider eating at home a realistic alternative.[B] Retail sales of food and drink in Europe's largest markets are at a standstill, leaving European grocery retailers hungry for opportunities to grow. Most leading retailers have already tried e-commerce, with limited success, and expansion abroad. But almost all have ignored the big, profitable opportunity in their own backyard: the wholesale food and drink trade, which appears to be just the kind of market retailers need.[C] Will such variations bring about a change in the overall structure of the food and drink market? Definitely not. The functioning of the market is based on flexible trends dominated by potential buyers. In other words, it is up to the buyer, rather than the seller, to decide what to buy .At any rate, this change will ultimately be acclaimed by an ever-growing number of both domestic and international consumers, regardless of how long the current consumer pattern will take hold.[D] All in all, this clearly seems to be a market in which big retailers could profitably apply their scale, existing infrastructure and proven skills in the management of product ranges, logistics, and marketing intelligence. Retailers that master the intricacies of wholesaling in Europe may well expect to rake in substantial profits thereby. At least, that is how it looks as a whole. Closer inspection reveals important differences among the biggest national markets, especially in their customer segments and wholesale structures, as well as the competitive dynamics of individual food and drink categories. Big retailers must understand these differences before they can identify the segments of European wholesaling in which their particular abilities might unseat smaller but entrenched competitors. New skills and unfamiliar business models are needed too.[E] Despite variations in detail, wholesale markets in the countries that have been closely examined—France, Germany, Italy, and Spain—are made out of the same building blocks. Demand comes mainly from two sources: independent mom-and-pop grocery stores which, unlike large retail chains, are two small to buy straight from producers, and food service operators that cater to consumers when they don't eat at home. Such food service operators range from snack machines to large institutional catering ventures, but most of these businesses are known in the trade as "horeca": hotels, restaurants, and cafes. Overall, Europe's wholesale market for food and drink is growing at the same sluggish pace as the retail market, but the figures, when added together, mask two opposing trends.[F] For example, wholesale food and drink sales come to $268 billion in France, Germany, Italy, Spain, and the United Kingdom in 2000—more than 40 percent of retail sales. Moreover, average overall margins are higher in wholesale than in retail; wholesale demand from the food service sector is growing quickly as more Europeans eat out more often; and changes in the competitive dynamics of this fragmented industry are at last making it feasible for wholesalers to consolidate.[G] However, none of these requirements should deter large retailers (and even some large good producers and existing wholesalers) from trying their hand, for those that master the intricacies of wholesaling in Europe stand to reap considerable gains.Coinciding with the groundbreaking theory of biological evolution proposed by British naturalist Charles Darwin in the 1860s, British social philosopher Herbert Spencer put forward his own theory of biological and cultural evolution. Spencer argued that all worldly phenomena, including human societies, changed over time, advancing toward perfection.41.____________.American social scientist Lewis Henry Morgan introduced another theory of cultural evolution in the late 1800s. Morgan, along with Tylor, was one of the founders of modern anthropology. In his work, he attempted to show how all aspects of culture changed together in the evolution of societies.42._____________.In the early 1900s in North America, German-born American anthropologist Franz Boas developed a new theory of culture known as historical particularism. Historical particularism, which emphasized the uniqueness of all cultures, gave new direction to anthropology. 43._____________.Boas felt that the culture of any society must be understood as the result of a unique history and not as one of many cultures belonging to a broader evolutionary stage or type of culture. 44._______________.Historical particularism became a dominant approach to the study of culture in American anthropology, largely through the influence of many students of Boas. But a number of anthropologists in the early 1900s also rejected the particularist theory of culture in favor of diffusionism. Some attributed virtually every important cultural achievement to the inventions of a few, especially gifted peoples that, according to diffusionists, then spread to other cultures. 45.________________.Also in the early 1900s, French sociologist Émile Durkheim developed a theory of culture that would greatly influence anthropology. Durkheim proposed that religious beliefs functioned to reinforce social solidarity. An interest in the relationship between the function of society and culture—known as functionalism—became a major theme in European, and especially British, anthropology.[A] Other anthropologists believed that cultural innovations, such as inventions, had a single origin and passed from society to society. This theory was known as diffusionism.[B] In order to study particular cultures as completely as possible, Boas became skilled in linguistics, the study of languages, and in physical anthropology, the study of human biology and anatomy.[C] He argued that human evolution was characterized by a struggle he called the "survival of the fittest," in which weaker races and societies must eventually be replaced by stronger, more advanced races and societies.[D] They also focused on important rituals that appeared to preserve a people's social structure, such as initiation ceremonies that formally signify children's entrance into adulthood.[E] Thus, in his view, diverse aspects of culture, such as the structure of families, forms of marriage, categories of kinship, ownership of property, forms of government, technology, and systems of food production, all changed as societies evolved.[F] Supporters of the theory viewed as a collection of integrated parts that work together to keep a society functioning.[G] For example, British anthropologists Grafton Elliot Smith and W. J. Perry incorrectly suggested, on the basis of inadequate information, that farming, pottery making, and metallurgy all originated in ancient Egypt and diffused throughout the world. In fact, all of these cultural developments occurred separately at different times in many parts of the world.The time for sharpening pencils, arranging your desk, and doing almost anything else instead of writing has ended. The first draft will appear on the page only if you stop avoiding the inevitable and sit, stand up, or lie down to write. (41) Be flexible. Your outline should smoothly conduct you from one point to the next, but do not permit it to railroad you. If a relevant and important idea occurs to you now, work it into the draft. (42) Grammar, punctuation, and spelling can wait until you revise. Concentrate on what you are saying. Good writing most often occurs when you are in hot pursuit of an idea rather than in a nervous search for errors.(43) Your pages will be easier to keep track of that way, and, if you have to clip a paragraph to place it elsewhere, you will not lose any writing on the other side.If you are working on a word processor, you can take advantage of its capacity to make additions and deletions as well as move entire paragraphs by making just a few simple keyboard commands. Some software programs can also check spelling and certain grammatical elements in your writing. (44) These printouts are also easier to read than the screen when you work on revisions.Once you have a first draft on paper, you can delete material that is unrelated to your thesis and add material necessary to illustrate your points and make your paper convincing. The student who wrote ―The A & P as a State of Mind‖ wisely dropped a paragraph that questioned whether Sammy displays chauvinistic attitudes toward women. (45) Remember that your initial draft is only that. You should go through the paper many times – and then again – working to substantiate and clarify your ideas. You may even end up with several entire versions of the paper. Rewrite. The sentences within each paragraph should be related to a single topic. Transitions should connect one paragraph to the next so that there are no abrupt or confusing shifts. Awkward or wordy phrasing or unclear sentences and paragraphs should be mercilessly poked and prodded into shape.[A] To make revising easier, leave wide margins and extra space between lines so that you can easily add words, sentences, and corrections. Write on only one side of the paper.[B] After you have clearly and adequately developed the body of your paper, pay particular attention to the introductory and concluding paragraphs. It’s probably best to write the introduction last, after you know precisely what you are introducing. Concluding paragraphs demand equal attention because they leave the reader with a final impression.[C] It’s worth remembering, however, that though a clean copy fresh off a printer may look terrific, it will read only as well as the thinking and writing that have gone into it. Many writers prudently store their data on disks and print their pages each time they finish a draft to avoid losing any material because of power failures or other problems.[D] It makes no difference how you write, just so you do. Now that you have developed a topic into a tentative thesis, you can assemble your notes and begin to flesh out whatever outline you have made.[E] Although this is an interesting issue, it has nothing to do with the thesis, which explains how the setting influences Sammy’s decision to quit his job. Instead of including that paragraph, she added one that described Lengel’s crabbed response to the girls so that she could lead up to the A & P ―policy‖ he enforces.[F] In the final paragraph about the significan ce of the setting in ―A & P,‖ the student brings together the reasons Sammy quit his job by referring to his refusal to accept Lengel’s store policies.[G] By using the first draft as a means of thinking about what you want to say, you will very likely discover more than your notes originally suggested. Plenty of good writers don’t use outlines at all but discover ordering principles as they write. Do not attempt to compose a perfectly correct draft the first time around.。
Dark Matter in the Solar System
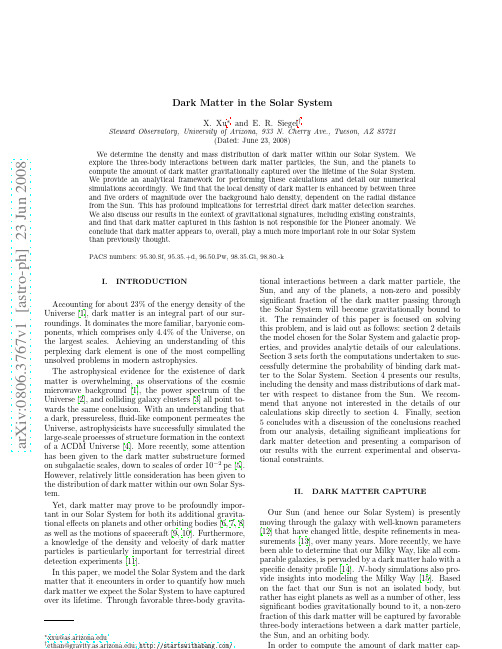
I.
INTRODUCTION
Accounting for about 23% of the energy density of the Universe [1], dark matter is an integral part of our surroundings. It dominates the more familiar, baryonic components, which comprises only 4.4% of the Universe, on the largest scales. Achieving an understanding of this perplexing dark element is one of the most compelling unsolved problems in modern astrophysics. The astrophysical evidence for the existence of dark matter is overwhelming, as observations of the cosmic microwave background [1], the power spectrum of the Universe [2], and colliding galaxy clusters [3] all point towards the same conclusion. With an understanding that a dark, pressureless, fluid-like component permeates the Universe, astrophysicists have successfully simulated the large-scale processes of structure formation in the context of a ΛCDM Universe [4]. More recently, some attention has been given to the dark matter substructure formed on subgalactic scales, down to scales of order 10−2 pc [5]. However, relatively little consideration has been given to the distribution of dark matter within our own Solar System. Yet, dark matter may prove to be profoundly important in our Solar System for both its additional gravitational effects on planets and other orbiting bodies [6, 7, 8] as well as the motions of spacecraft [9, 10]. Furthermore, a knowledge of the density and velocity of dark matter particles is particularly important for terrestrial direct detection experiments [11]. In this paper, we model the Solar System and the dark matter that it encounters in order to quantify how much dark matter we expect the Solar System to have captured over its lifetime. Through favorable three-body gravita-
暗物质英文介绍
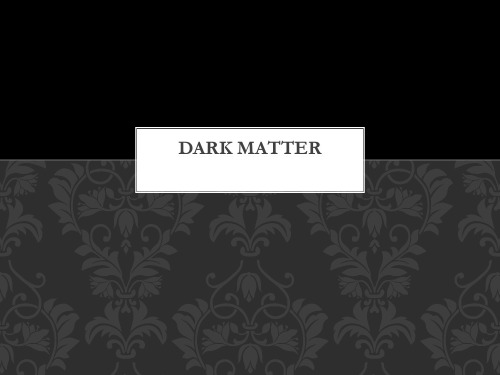
Detection
Direct detection experiments
Indirect detection experiments
DETECTION
If the dark matter within our galaxy is made up of Weakly Interacting Massive Particles (WIMPs), then thousands of WIMPs must pass through every square centimeter of the Earth each second.[84][85] There are many experiments currently running, or planned, aiming to test this hypothesis by searching for WIMPs. Although WIMPs are the historically more popular dark matter candidate for searches,[9] there are experiments searching for other particle candidates; the Axion Dark Matter eXperiment (ADMX) is currently searching for the dark matter axion, a well-motivated and constrained dark matter source. It is also possible that dark matter consists of very heavy hidden sector particles which only interact with ordinary matter via gravity.
Investigating the Nature of Dark Matter
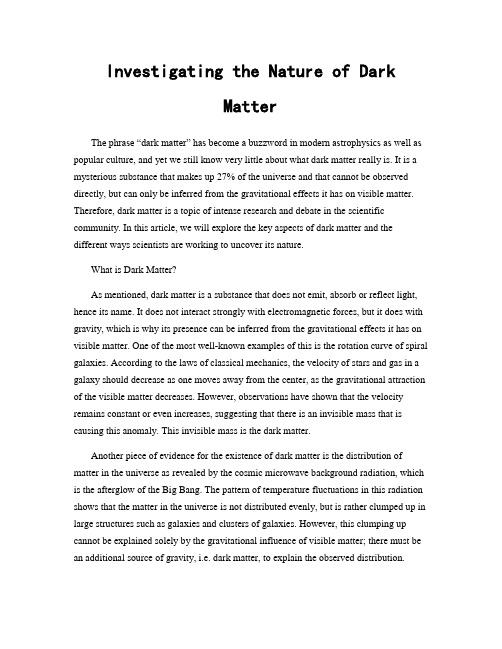
Investigating the Nature of DarkMatterThe phrase “dark matter” has become a buzzword in modern astrophysics as well as popular culture, and yet we still know very little about what dark matter really is. It is a mysterious substance that makes up 27% of the universe and that cannot be observed directly, but can only be inferred from the gravitational effects it has on visible matter. Therefore, dark matter is a topic of intense research and debate in the scientific community. In this article, we will explore the key aspects of dark matter and the different ways scientists are working to uncover its nature.What is Dark Matter?As mentioned, dark matter is a substance that does not emit, absorb or reflect light, hence its name. It does not interact strongly with electromagnetic forces, but it does with gravity, which is why its presence can be inferred from the gravitational effects it has on visible matter. One of the most well-known examples of this is the rotation curve of spiral galaxies. According to the laws of classical mechanics, the velocity of stars and gas in a galaxy should decrease as one moves away from the center, as the gravitational attraction of the visible matter decreases. However, observations have shown that the velocity remains constant or even increases, suggesting that there is an invisible mass that is causing this anomaly. This invisible mass is the dark matter.Another piece of evidence for the existence of dark matter is the distribution of matter in the universe as revealed by the cosmic microwave background radiation, which is the afterglow of the Big Bang. The pattern of temperature fluctuations in this radiation shows that the matter in the universe is not distributed evenly, but is rather clumped up in large structures such as galaxies and clusters of galaxies. However, this clumping up cannot be explained solely by the gravitational influence of visible matter; there must be an additional source of gravity, i.e. dark matter, to explain the observed distribution.Moreover, measurements of the large-scale structure of the universe, such as the distribution of galaxies and galaxy clusters, also point to the existence of dark matter.What is Dark Matter Made of?Despite its importance in shaping the structure of the universe, the identity of dark matter remains unknown. There are several hypotheses about what dark matter might be made of, but none of them has been conclusively proven yet. One popular hypothesis is that dark matter is composed of weakly interacting massive particles (WIMPs), which are hypothetical particles that would interact with normal matter only through the weak nuclear force and gravity. The idea is that WIMPs were produced in the early universe when it was hot and dense, and have been moving around freely ever since. If they collide with normal matter, they would transfer some of their energy and momentum, producing detectable signals. In fact, several experiments have been designed to detect WIMP interactions, such as the Large Underground Xenon (LUX) experiment and the Super Cryogenic Dark Matter Search (SuperCDMS).Another hypothesis is that dark matter is made of axions, which are theoretical particles that were originally proposed to explain a different problem in physics, the strong CP problem. The idea is that axions would be very light and weakly interacting, making them difficult to detect, but would still affect the motion of galaxies and other cosmic structures. The Axion Dark Matter eXperiment (ADMX) is currently searching for evidence of axions in a laboratory at the University of Washington.A third hypothesis is that dark matter is composed of primordial black holes, which are black holes that were formed by the collapse of a density fluctuation in the early universe. The idea is that these black holes could have a mass range that would make them more likely to be dark matter, and that their interactions with normal matter could produce observable effects. However, this hypothesis is less favored by most researchers, as the formation and stability of such black holes would require very specific conditions.ConclusionDespite decades of research, the nature of dark matter remains one of the most intriguing and elusive topics in astrophysics. It remains a theoretical construct that cannot be directly observed, but its effects on the motion and structure of the cosmos are undeniable. Researchers are continuing to study dark matter using a variety of tools and techniques, from telescopes that measure gravitational lensing to underground experiments that look for WIMP interactions. The hope is that someday we will finally be able to unravel the mystery of what dark matter is made of, and in doing so, gain a better understanding of the universe and our place in it.。
Dark Matter
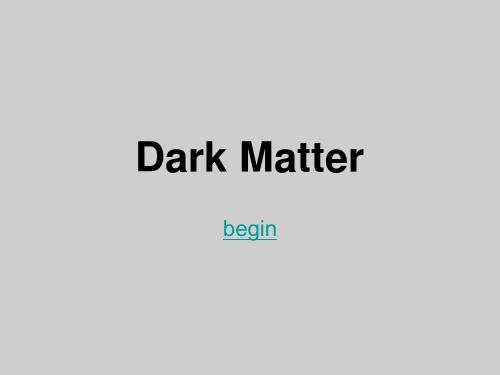
Mass / Energy Density
Scientists have measured the density of the universe by studying clusters of galaxies. We can call it either mass density or energy density. Einstein proved that mass and energy are the same with his equation E = mc2. He’s also the guy to blame for this curved space-time stuff. (learn more)
But . . .
But outer stars do not rotate correctly! If gravity causes galaxies to rotate, as we assume it does, then outer stars should behave much like the planets of our solar system. Inner planets rotate faster and outer planets rotate slower. This is called Keplerian motion. (learn more) In galaxies, however, both inner and outer stars rotate at about the same speed.
The Universe
Cosmologists study the birth and death of the universe. They also study its properties including its shape. Recent observation indicate that the universe is flat.
The physics of dark matter and its properties
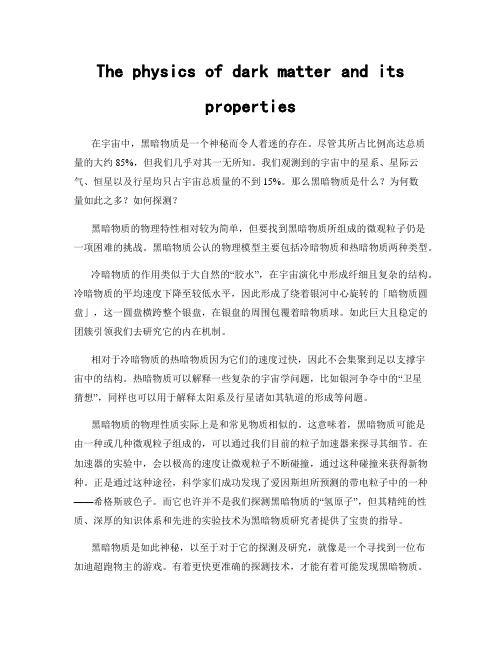
The physics of dark matter and itsproperties在宇宙中,黑暗物质是一个神秘而令人着迷的存在。
尽管其所占比例高达总质量的大约85%,但我们几乎对其一无所知。
我们观测到的宇宙中的星系、星际云气、恒星以及行星均只占宇宙总质量的不到15%。
那么黑暗物质是什么?为何数量如此之多?如何探测?黑暗物质的物理特性相对较为简单,但要找到黑暗物质所组成的微观粒子仍是一项困难的挑战。
黑暗物质公认的物理模型主要包括冷暗物质和热暗物质两种类型。
冷暗物质的作用类似于大自然的“胶水”,在宇宙演化中形成纤细且复杂的结构。
冷暗物质的平均速度下降至较低水平,因此形成了绕着银河中心旋转的「暗物质圆盘」,这一圆盘横跨整个银盘,在银盘的周围包覆着暗物质球。
如此巨大且稳定的团簇引领我们去研究它的内在机制。
相对于冷暗物质的热暗物质因为它们的速度过快,因此不会集聚到足以支撑宇宙中的结构。
热暗物质可以解释一些复杂的宇宙学问题,比如银河争夺中的“卫星猜想”,同样也可以用于解释太阳系及行星诸如其轨道的形成等问题。
黑暗物质的物理性质实际上是和常见物质相似的。
这意味着,黑暗物质可能是由一种或几种微观粒子组成的,可以通过我们目前的粒子加速器来探寻其细节。
在加速器的实验中,会以极高的速度让微观粒子不断碰撞,通过这种碰撞来获得新物种。
正是通过这种途径,科学家们成功发现了爱因斯坦所预测的带电粒子中的一种——希格斯玻色子。
而它也许并不是我们探测黑暗物质的“氢原子”,但其精纯的性质、深厚的知识体系和先进的实验技术为黑暗物质研究者提供了宝贵的指导。
黑暗物质是如此神秘,以至于对于它的探测及研究,就像是一个寻找到一位布加迪超跑物主的游戏。
有着更快更准确的探测技术,才能有着可能发现黑暗物质。
DOMINO的黑暗物质观测是对现有探测方式的重大突破,不仅如此,有关黑暗物质的实验裂变也已成为目前物理学中最火热的研究领域之一。
总的来说,黑暗物质的探寻和研究是相当具有挑战性的,要想达到理想的研究结果,需要一个有才华的科研团队,也需要更加精密的实验方法和仪器来进行探测,一次次的试验和尝试,才有可能让我们找到这个宇宙深处的谜团。
科学英语:黑洞可能是研究暗物质理想的实验场所
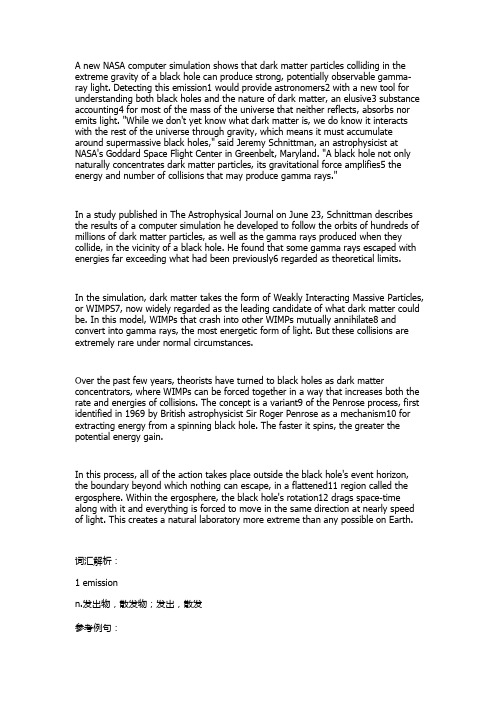
A new NASA computer simulation shows that dark matter particles colliding in the extreme gravity of a black hole can produce strong, potentially observable gamma-ray light. Detecting this emission1 would provide astronomers2 with a new tool forunderstanding both black holes and the nature of dark matter, an elusive3 substance accounting4 for most of the mass of the universe that neither reflects, absorbs nor emits light. "While we don't yet know what dark matter is, we do know it interacts with the rest of the universe through gravity, which means it must accumulate around supermassive black holes," said Jeremy Schnittman, an astrophysicist at NASA's Goddard Space Flight Center in Greenbelt, Maryland. "A black hole not only naturally concentrates dark matter particles, its gravitational force amplifies5 the energy and number of collisions that may produce gamma rays."In a study published in The Astrophysical Journal on June 23, Schnittman describes the results of a computer simulation he developed to follow the orbits of hundreds of millions of dark matter particles, as well as the gamma rays produced when they collide, in the vicinity of a black hole. He found that some gamma rays escaped with energies far exceeding what had been previously6 regarded as theoretical limits.In the simulation, dark matter takes the form of Weakly Interacting Massive Particles, or WIMPS7, now widely regarded as the leading candidate of what dark matter could be. In this model, WIMPs that crash into other WIMPs mutually annihilate8 and convert into gamma rays, the most energetic form of light. But these collisions are extremely rare under normal circumstances.Over the past few years, theorists have turned to black holes as dark matter concentrators, where WIMPs can be forced together in a way that increases both the rate and energies of collisions. The concept is a variant9 of the Penrose process, first identified in 1969 by British astrophysicist Sir Roger Penrose as a mechanism10 for extracting energy from a spinning black hole. The faster it spins, the greater the potential energy gain.In this process, all of the action takes place outside the black hole's event horizon, the boundary beyond which nothing can escape, in a flattened11 region called the ergosphere. Within the ergosphere, the black hole's rotation12 drags space-time along with it and everything is forced to move in the same direction at nearly speed of light. This creates a natural laboratory more extreme than any possible on Earth.词汇解析:1 emissionn.发出物,散发物;发出,散发参考例句:Rigorous measures will be taken to reduce the total pollutant emission.采取严格有力措施,降低污染物排放总量。
The Science of Dark Matter and Its Discovery
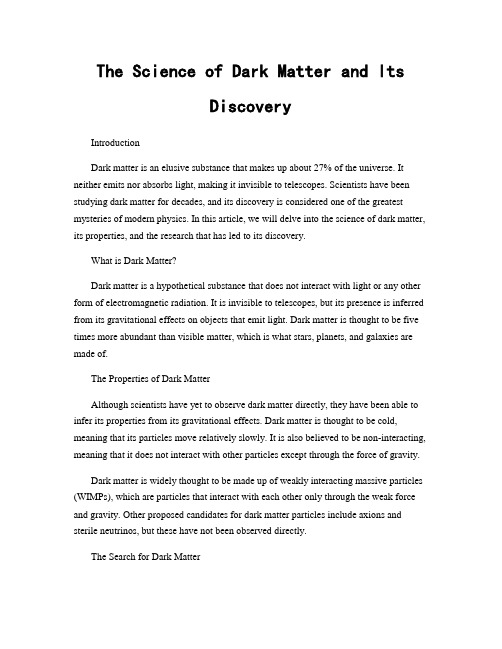
The Science of Dark Matter and ItsDiscoveryIntroductionDark matter is an elusive substance that makes up about 27% of the universe. It neither emits nor absorbs light, making it invisible to telescopes. Scientists have been studying dark matter for decades, and its discovery is considered one of the greatest mysteries of modern physics. In this article, we will delve into the science of dark matter, its properties, and the research that has led to its discovery.What is Dark Matter?Dark matter is a hypothetical substance that does not interact with light or any other form of electromagnetic radiation. It is invisible to telescopes, but its presence is inferred from its gravitational effects on objects that emit light. Dark matter is thought to be five times more abundant than visible matter, which is what stars, planets, and galaxies are made of.The Properties of Dark MatterAlthough scientists have yet to observe dark matter directly, they have been able to infer its properties from its gravitational effects. Dark matter is thought to be cold, meaning that its particles move relatively slowly. It is also believed to be non-interacting, meaning that it does not interact with other particles except through the force of gravity.Dark matter is widely thought to be made up of weakly interacting massive particles (WIMPs), which are particles that interact with each other only through the weak force and gravity. Other proposed candidates for dark matter particles include axions and sterile neutrinos, but these have not been observed directly.The Search for Dark MatterThe search for dark matter has been ongoing for several decades. One of the most promising methods for detecting dark matter involves looking for the energetic particles that result from the annihilation of dark matter particles. This method is called indirect detection and involves searching for gamma rays, neutrinos, or cosmic rays that are produced by the decay or annihilation of dark matter particles.Another way to detect dark matter is through the direct detection method, which involves looking for the recoil of atomic nuclei in a detector after they have been struck by dark matter particles. This method requires a sophisticated detector that can detect even the slightest signal. Several experiments are currently underway to detect dark matter particles using these methods.Discovery of Dark MatterThe discovery of dark matter can be traced back to the 1930s when Swiss astronomer Fritz Zwicky observed that the visible matter in the Coma cluster of galaxies was not enough to hold the cluster together. He hypothesized the presence of invisible matter that was holding the cluster together, which he called dark matter.Over the years, other scientists have provided evidence for the existence of dark matter. In the 1970s, Vera Rubin and Kent Ford studied the rotation curves of galaxies and found that the observed mass could not account for the observed rotation speeds. They concluded that there must be more mass in the form of dark matter that was holding the galaxies together.More recently, the European Space Agency’s Planck satellite produced a detailed map of the cosmic microwave background radiation, which is thought to be leftover radiation from the Big Bang. The map provided strong evidence for the existence of dark matter and its abundance in the universe.ConclusionThe discovery of dark matter is one of the most exciting and challenging areas of modern physics. Scientists continue to search for dark matter using a variety of methods, including indirect and direct detection. Although dark matter has yet to be observeddirectly, its presence and properties can be inferred from its gravitational effects on visible matter. As we continue to unravel the mysteries of dark matter, we are sure to gain new insights and a deeper understanding of the universe we inhabit.。
暗物质(精)

the different number of protons, neutrons Jiu Ge together form various chemical elements, and finally form the universe galaxies. With the spread of the explosion outcome, temperature, pressure and density, gradually reduce total quality 95.6% residual quarks and gluons can neither mutual combination also cannot constitute the stable particles, and only in pre-columri prior group continued expands outward diffusion. Only the quarks and gluons entwined form the protons and neutrons particle scale can present strong forces; Precolumri prior quarks only presents pure weak mutual attraction, and its physical properties and have strong forces quarks widely divergent, neither absorb light nor reflected light, thus formed the unierse.
Cosmic explosions produce a lot of quarks - gluons plasma, in explosion of high temperature and high pressure, high density, high shear stress condition, a few gluons vortex formed the quarks winding up protons and neutrons, some negative quantum conservation gluons vortex group constituted from stable particles - electronic, The universe of protons, neutrons and electrons is equal to the sum of the quality of the total weight of the universe just 4.4 per cent, which could determine dark matter in the universe and dark energy and explicit physical quality ratio is a career-high 21.6 the cosmological constant. As the temperature further reduce, in chaos by protons, neutrons and electrons constitute the sensible substance in the electromagnetic force, weak forces and gravitation under the action of gradually together, In the basic particle of small scale strong forces not only to maintain the stability of quarks and gluons combination,
The mysteries of the universe Dark matter

The mysteries of the universe DarkmatterDark matter is one of the most intriguing mysteries of the universe,captivating the curiosity of scientists and enthusiasts alike. This enigmatic substance makes up approximately 27% of the universe, yet its presence remains largely elusive and perplexing. Unlike ordinary matter, dark matter does not emit, absorb, or reflect light, making it invisible and undetectable through traditional means. Its existence is inferred through its gravitational effects on visible matter, such as galaxies and galaxy clusters. Despite decades of research and numerous experiments, the true nature of dark matter continues to elude us,leaving us with more questions than answers. The search for dark matter has led scientists to explore various theories and hypotheses in an attempt to unravel its secrets. One prevailing theory posits that dark matter consists of exoticparticles that interact weakly with ordinary matter, known as Weakly Interacting Massive Particles (WIMPs). These hypothetical particles are believed to haveplayed a crucial role in the formation and evolution of the universe, influencing the distribution of matter on cosmic scales. While numerous experiments have been conducted to detect WIMPs, none have yielded conclusive evidence of their existence, adding to the mystery surrounding dark matter. Another intriguing aspect of dark matter is its role in shaping the structure of the universe. Through its gravitational influence, dark matter acts as a cosmic scaffolding, guiding the formation of galaxies and galaxy clusters. Without dark matter, the universe as we know it would look vastly different, with galaxies failing to form and cosmic structures remaining diffuse and disorganized. Understanding the roleof dark matter in cosmic evolution is essential for unraveling the mysteries ofthe universe and gaining insights into its origins and ultimate fate. The questto unravel the mysteries of dark matter has spurred the development of innovative technologies and experimental techniques aimed at detecting its elusive presence. From deep underground laboratories to space-based observatories, scientists are employing a wide range of tools and instruments to search for signs of dark matter. One such experiment is the Large Hadron Collider (LHC) at CERN, which aims torecreate the conditions present in the early universe to study the fundamental particles and forces that govern the cosmos. While the LHC has not yet detected dark matter directly, it has provided valuable insights into the nature of the universe and the fundamental forces that shape it. Despite the challenges and uncertainties surrounding dark matter, the quest to unlock its secrets continues to inspire and captivate scientists and enthusiasts alike. The tantalizing prospect of unraveling one of the universe's greatest mysteries drives researchers to push the boundaries of knowledge and explore new frontiers in physics and cosmology. As we delve deeper into the nature of dark matter, we are not only expanding our understanding of the universe but also challenging our perceptions of reality and the fundamental laws that govern it. The search for dark matter reminds us of the boundless wonders of the cosmos and the endless possibilities that lie beyond our current understanding.。
The Role of Dark Matter in the Universe

The Role of Dark Matter in theUniverseIntroductionThe universe is full of mysteries, and one of the biggest enigmas is the presence of dark matter. Dark matter is a hypothetical type of matter that cannot be observed directly. This invisible substance is believed to make up around 85% of the matter in the universe, yet it remains one of the most elusive components of our cosmos. In this article, we will explore the role of dark matter in the universe and why it is so important.What is Dark Matter?Dark matter is a form of matter that does not emit, absorb, or reflect light, making it invisible to telescopes and other instruments. It cannot be detected directly, but its presence can be inferred through its effects on other objects, such as stars and galaxies. Scientists believe that dark matter interacts only through the force of gravity, which means that it does not interact with light or other forms of electromagnetic radiation.What is the Role of Dark Matter in the Universe?Dark matter has a significant role in the universe as it plays a crucial part in the formation and evolution of galaxies. The gravity of dark matter is believed to be responsible for the formation of large structures in the universe, including galaxy clusters and superclusters.Dark matter is also believed to have played a significant role in the formation of the earliest galaxies in the universe. The Big Bang theory suggests that the universe began with an explosion that created a hot, dense soup of particles. As the universe cooled, these particles came together to form the first atoms, which then coalesced into galaxies. Dark matter is thought to have collected together in the early universe, forming the framework of the first galaxies.The presence of dark matter also affects the movement of stars in galaxies. When astronomers study the motions of stars, they notice that the stars' velocities do not match what would be expected based on the visible matter alone. This discrepancy suggests that there is some invisible matter, such as dark matter, that is generating a gravitational force, which affects the stars' movements.Dark matter also has a vital role in the universe's evolution by slowing down the rate of expansion. The universe is continually expanding, but the presence of dark matter slows down this expansion by exerting a gravitational force on visible matter. This force can act as a counterbalance to the outward force of expansion, helping to keep galaxies and other structures from flying apart.Why is Dark Matter Important?Even though dark matter cannot be observed directly, its effects on visible matter allow scientists to study its properties and understand its role in the universe's evolution. The existence of dark matter also provides evidence for the existence of other dimensions and the possibility of new physics beyond the standard model.Scientists are continually searching for ways to detect dark matter, such as searching for interactions between dark matter particles and ordinary matter or using indirect methods such as studying the cosmic microwave background radiation. The discovery of dark matter would not only help us to understand the universe's structure and evolution but could also provide insights into the nature of dark energy, which is another mysterious and elusive component of the universe.ConclusionDark matter remains one of the most significant mysteries of the universe, and its role in the formation and evolution of galaxies is crucial. Its presence can be inferred through its effects on visible matter, and scientists are continually searching for ways to detect it. Understanding dark matter's properties and interactions could help us unlock even more secrets of the universe and alter our understanding of the cosmos.。
Dark matter and structure formation a review
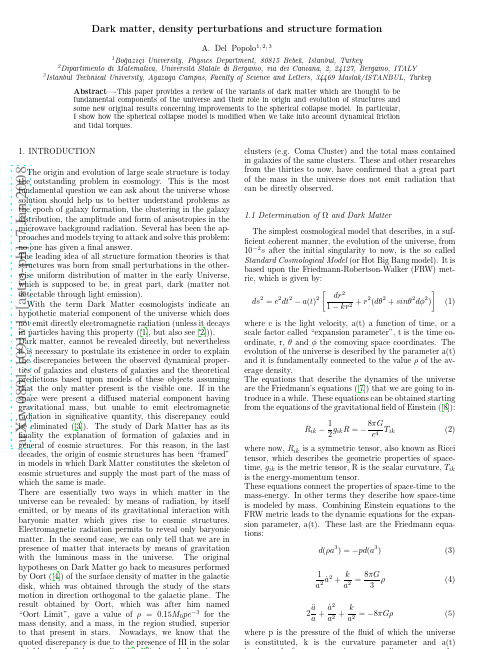
a r X i v :0801.1091v 1 [a s t r o -p h ] 7 J a n 2008Dark matter,density perturbations and structure formationA.Del Popolo 1,2,31Bo ˘g azi ¸c i University,Physics Department,80815Bebek,Istanbul,Turkey2Dipartimento di Matematica,Universit`a Statale di Bergamo,via dei Caniana,2,24127,Bergamo,ITALY 3Istanbul Technical University,Ayazaga Campus,Faculty of Science and Letters,34469Maslak/ISTANBUL,TurkeyAbstract —-This paper provides a review of the variants of dark matter which are thought to be fundamental components of the universe and their role in origin and evolution of structures and some new original results concerning improvements to the spherical collapse model.In particular,I show how the spherical collapse model is modified when we take into account dynamical friction and tidal torques.1.INTRODUCTIONThe origin and evolution of large scale structure is today the outstanding problem in cosmology.This is the most fundamental question we can ask about the universe whose solution should help us to better understand problems as the epoch of galaxy formation,the clustering in the galaxy distribution,the amplitude and form of anisotropies in the microwave background radiation.Several has been the ap-proaches and models trying to attack and solve this problem:no one has given a final answer.The leading idea of all structure formation theories is that structures was born from small perturbations in the other-wise uniform distribution of matter in the early Universe,which is supposed to be,in great part,dark (matter not detectable through light emission).With the term Dark Matter cosmologists indicate an hypothetic material component of the universe which does not emit directly electromagnetic radiation (unless it decays in particles having this property ([1],but also see [2])).Dark matter,cannot be revealed directly,but nevertheless it is necessary to postulate its existence in order to explain the discrepancies between the observed dynamical proper-ties of galaxies and clusters of galaxies and the theoretical predictions based upon models of these objects assuming that the only matter present is the visible one.If in the space were present a diffused material component having gravitational mass,but unable to emit electromagnetic radiation in significative quantity,this discrepancy could be eliminated ([3]).The study of Dark Matter has as its finality the explanation of formation of galaxies and in general of cosmic structures.For this reason,in the last decades,the origin of cosmic structures has been “framed”in models in which Dark Matter constitutes the skeleton of cosmic structures and supply the most part of the mass of which the same is made.There are essentially two ways in which matter in the universe can be revealed:by means of radiation,by itself emitted,or by means of its gravitational interaction with baryonic matter which gives rise to cosmic structures.Electromagnetic radiation permits to reveal only baryonic matter.In the second case,we can only tell that we are in presence of matter that interacts by means of gravitation with the luminous mass in the universe.The original hypotheses on Dark Matter go back to measures performed by Oort ([4])of the surface density of matter in the galactic disk,which was obtained through the study of the stars motion in direction orthogonal to the galactic plane.The result obtained by Oort,which was after him named “Oort Limit”,gave a value of ρ=0.15M 0pc −3for the mass density,and a mass,in the region studied,superior to that present in stars.Nowadays,we know that the quoted discrepancy is due to the presence of HI in the solarclusters (a Cluster)and the total mass contained in galaxies of the same clusters.These and other researches from the thirties to now,have confirmed that a great part of the mass in the universe does not emit radiation that can be directly observed.1.1Determination of Ωand Dark MatterThe simplest cosmological model that describes,in a suf-ficient coherent manner,the evolution of the universe,from 10−2s after the initial singularity to now,is the so called Standard Cosmological Model (or Hot Big Bang model).It is based upon the Friedmann-Robertson-Walker (FRW)met-ric,which is given by:ds 2=c 2dt 2−a (t )2dr 22g ik R =−8πGa 2˙a 2+k3ρ(4)2¨a a 2+k2 the components of the today universe are galaxies.If weassume that galaxies motion satisfy Weyl([9])postulate,the velocity vector of a galaxy is given by u i=(1,0,0,0),and then the system behaves as a system made of dust forwhich we have p=0.Only two of the three Friedmannequations are independent,because thefirst connectsdensity,ρto the expansion parameter a(t).The characterof the solutions of these equations depends on the valueof the curvature parameter,k,which is also determinedby the initial conditions by means of Eq. 3.The solutionto the equations now written shows that ifρis largerthanρc=3H2ρc .In this case,theconditionΩ=1corresponds to k=0,Ω<1corresponds to k=−1,andΩ>1corresponds to k=1.1The value ofΩcan be calculated in several ways.The most common methods are the dynamical methods,in which the effects of gravity are used,and kinematics methods sensible to the evolution of the scale factor and to the space-time geometry.The results obtained forΩwith these different methods are summarized in the following.Dynamical methods:(a)Rotation curves:The contribution of spiral galaxies to the density in the universe is calculated by using their rotation curves and the third Kepler ing the last it is possible to obtain the mass of a spiral galaxy from the equation:M(r)=v2r/G(6) where v is the velocity of a test particle at a distance r from the center and M(r)is the mass internal to the circular orbit of the particle.In order to determine the mass M is necessary to have knowledge of the term v2in Eq.(6)and this can be done from the study of the rotation curves through the21cm line of HI.Rotation curves of galaxies are characterized by a peak reached at distances of some Kpcs and a behavior typicallyflat for the regions at distance larger than that of the peak.A peculiarity is that the expected Keplerian fall is not observed.This result is consistent with extended haloes containing masses till10times the galactic mass observed in the optical ([10]).The previous result is obtained assuming that the halo mass obtained with this method is distributed in a spherical region so that we can use Eq.(6)and that we neglect the tidal interaction with the neighboring galaxies which tend to produce an expansion of the halo.After M and the luminosity of a series of elliptical galaxies is determined,the contribution to the density of the universeL>ℓwhereℓis the luminosity per unit volume due to galaxies and can be obtained from the galactic luminosity functionφ(L)dL,which describes the number of galaxies per Mpc3and luminosity range L,L+dL.The value that is usually assumed forℓis ℓ=2.4h108L bo Mpc−3.The arguments used lead to a value ofΩg for the luminous parts of spiral galaxies ofΩg≤0.01, while for haloesΩh≥0.03−0.1.The result shows that the halo mass is noteworthy larger than the galactic mass observable in the optical([11]).(b)Virial theorem:In the case of non spiral galaxies and clusters,the mass can be obtained using the virial theorem2T+V=0,withT∼=3c≈Ω0.6λρ(9) ([13]).Then given the overdensityδρρcan be ob-tained from the overdensity of galaxiesδn gρ=δn g3 (d)Kinematic methods:These methods are based upon the use of relations be-tween physical quantities dependent on cosmological param-eters.An example of those relations is the relation luminos-ity distance-redshift:H0d L=z+1F the luminosity distance,L the absolute luminos-ity,and F theflux.By means of the relations luminosity-redshift,angle-redshift,number of objects-redshift,it is possible to determine the parameter of deceleration q0=−¨a0a0=100hkm/Mpcs are the scale factor and the Hubble constant,nowadays).At the same time q0is connected toΩby means of q0=Ω•Growth rate offlparisons of presentday structure withfluctuations at the last scatteringof the cosmic microwave background(CMB)or withhigh redshift objects of the young universe.The methods and current estimates are summarized in Table3.The estimates based on virialized objects typi-cally yield low values ofΩm∼0.2−0.3.The global mea-sures,large-scale structure and cosmicflows typically indi-cate higher valuesofΩm∼0.4−1.Bahcall et al.([17]),showed that the evolution of the number density of rich clusters of galaxies breaks the degen-eracy betweenΩ(the mass density ratio of the universe)and σ8(the normalization of the power spectrum),σ8Ω0.5≃0.5, that follows from the observed present-day abundance of rich clusters.The evolution of high-mass(Coma-like)clus-ters is strong inΩ=1,low-σ8models(such as the standard biased CDM model withσ8≃0.5),where the number den-sity of clusters decreases by a factor of∼103from z=0 to z≃0.5;the same clusters show only mild evolution in low-Ω,high-σ8models,where the decrease is a factor of ∼10.This diagnostic provides a most powerful constraint onΩ.Using observations of clusters to z≃0.5−1,the authors found only mild evolution in the observed cluster abundance,andΩ=0.3±0.1andσ8=0.85±0.15(for Λ=0models;forΩ+Λ=1models,Ω=0.34±0.13). ferreira et al.([18]),proposed an alternative method to estimate v12directly from peculiar velocity samples,which contain redshift-independent distances as well as galaxy red-shifts.In contrast to other dynamical measures which de-termineβ≡Ω0.6σ8,this method can provide an estimate of Ω0.6σ28for a range ofσ8whereΩis the cosmological density parameter,whileσ8is the standard normalization for the power spectrum of densityfluctuations.Melchiorri([19]),used the angular power spectrum of the Cosmic Microwave Background,measured during the North American testflight of the BOOMERANG experiment,to constrain the geometry of the universe.Within the class of Cold Dark Matter models,theyfind that the overall frac-tional energy density of the universe,Ω,is constrained to be0.85≤Ω≤1.25at the68%confidence level. Branchini([20]),compared the density and velocityfields as extracted from the Abell/ACO clusters to the corre-spondingfields recovered by the POTENT method from the Mark III peculiar velocities of galaxies.Quantitative comparisons within a volume containing∼12independent samples yieldβc≡Ω0.6/b c=0.22±0.08,where b c is the cluster biasing parameter at15h−1Mpc.If b c∼4.5,as in-dicated by the cluster correlation function,their result is consistent withΩ∼1.(f)Inflation:It is widely supposed that the very early universe experi-enced an era of inflation(see[21],[22],[13]).By‘inflation’one means that the scale factor has positive acceleration,¨a>0,corresponding to repulsive gravity and3p<−ρ. During inflation aH=˙a is increasing,so that comoving scales are leaving the horizon(Hubble distance)rather than entering it,and it is supposed that at the beginning of in-flation the observable universe was well within the horizon. The inflationary hypothesis is attractive because it holds out the possibility of calculating cosmological quantities, given the Lagrangian describing the fundamental interac-tions.The Standard Model,describing the interactions up to energies of order1T eV,is not viable in this context be-cause it does not permit inflation,but this should not be re-garded as a serious setback because it is universally agreed4mology.The nature of the required extension is not yet known,though it is conceivable that it could become known in the foreseeable future.But even without a specific model of the interactions(ie.,a specific Lagrangian),the inflation-ary hypothesis can still offer guidance about what to expect in cosmology.More dramatically,one can turn around the theory-to-observation sequence,to rule out otherwise rea-sonable models.The importance of inflation is connected to:a)the origin of density perturbations,which could origi-nate during inflation as quantumfluctuations,which be-come classical as they leave the horizon and remain so on re-entry.The original quantumfluctuations are of exactly the same type as those of the electromagneticfield,which give rise to the experimentally observed Casimir effect. b)One of the most dramatic and simple effects is that there is nofine-tuning of the initial value of the density parame-terΩ=8πρ/3m2P l H2.From the Friedmann equation,Ωis given byΩ−1=(K3An argument has been given forΩ0very close to1on the basis of effects on the cmb anisotropy from regions far outside the observable simplest one([21])invokes a scalarfield,termed the infla-tonfield.An alternative([23])is to invoke a modification of Einstein gravity,and combinations of the two mecha-nisms have also been proposed.During inflation however, the proposed modifications of gravity can be abolished by redefining the spacetime metric tensor,so that one recovers the scalarfield case.We focus on it for the moment,but modified gravity models will be included later in our survey of specific models.In comoving coordinates a homogeneous scalarfieldφwith minimal coupling to gravity has the equation of motion¨φ+3H˙φ+V′(φ)=0(13) Its energy density and pressure areρ=V+12˙φ2(15)If such afield dominatesρand p,the inflationary condition 3p<ρis achieved provided that thefield rolls sufficiently slowly,˙φ2<V(16)Practically all of the usually considered models of inflation satisfy three conditions.First,the motion of thefield is overdamped,so that the‘force’V′balances the‘friction term’3H˙φ,˙φ≃−116π V′38π8πV′′5 in which they are satisfied and we are adopting that nomen-clature here.Practically all of the usually considered modelsof inflation satisfy the slow-roll conditions more or less well.It should be noted that thefirst slow-roll condition is ona quite different footing from the other two,being a state-ment about the solution of thefield equation as opposed toa statement about the potential that defines this equation.What we are saying is that in the usually considered modelsone can show that thefirst condition is an attractor solu-tion,in a regime typically characterized by the other twoconditions,and that moreover reasonable initial conditionsonφwill ensure that this solution is achieved well beforethe observable universe leaves the horizon.It is importantto remember that there are strong observational limits forthe parameters previously introduced(e.g.ǫ,η).For ex-ample[27]studied the possible contribution of a stochasticgravitational wave background to the anisotropy of the cos-mic microwave background in cold and mixed dark matter(CDM and MDM)models.This contribution was testedagainst detections of CMB anisotropy at large and inter-mediate angular scales.The bestfit parameters(i.e.thosewhich maximize the likelihood)are(with95%confidence)n S=1.23+0.17−0.15andR(n S)=C T2π2f(n S)=2.4+3.4−2.2(23)wheref(n S)=Γ(3−n S)Γ(3+n SΓ2(4−n S2)(24)The previous constraintfixes the value ofǫas well that ofη2η=n s−1+2ǫ(25) Theyfind that by including the possibility of such back-ground in CMB data analysis it can drastically alter the conclusion on the remaining cosmological parameters.More stringent constraints on some of the previous parameters are given in section1.12.(h)Conclusions:We have seen the possible values ofΩusing different meth-ods.We have to add that Cosmologists are“attracted”by a value ofΩ0=1.This value ofΩis requested by infla-tionary theory.The previous data lead us to the following hypotheses:i)Ω0<0.12;in this case one can suppose that the uni-verse is fundamentally made of baryonic matter(black holes; Jupiters;white dwarfs).ii)Ω0>0.12;in this case in order to have aflat universe,it is necessary a non-baryonic component.Ωb=1is excluded by several reasons(see[28],[13].The remaining possibilities are:1)existence of a smooth component withΩ=0.8.The test of a smooth component can be done with kinematic methods.2)Existence of a cosmological term,absolutely smooth to whom correspond an energy densityρvac=Λsthe number of particles per unit comoving volume and we remember that n is the number density of species and s the entropy density, we obtain a contribution of the species to the actual density of the universe asΩh2=0.28Y(T f)(m6limits([13]).The solution to the problem was proposed by Peccei-Quinn in1977([36])in terms of a spontaneous sym-metry breaking scheme.To this symmetry breaking should be associated a Nambu-Goldstone boson:the axion.Theaxion mass ranges between10−12ev-1Gev.In cosmology there are two ranges of interest:10−6ev≤m a≤10−3ev; 3ev≤m a≤8ev.Axion production in the quoted range can originate due to a series of astrophysical processes([13])and several are the ways these particles can be detected. Nevertheless the effort of researchers expecially in USA, Japan and Italy,axions remain hypothetical particles. They are in any case the most important CDM candidates.In the following,I am going to speak about the basic ideas of structure formation.I shall write about density perturbations,their spectrum and evolution,about correla-tion functions and their time evolution,etc.1.3Origin of structuresObserving our universe,we notice a clear evidence of in-homogeneity when we consider small scales(Mpcs).In clus-ters density reaches values of103times larger than the av-erage density,and in galaxies it has values105larger than the average density([13]).If we consider scales larger than 102Mpcs universe appears isoptric as it is observed in the radio-galaxies counts,in CMBR,in the X background([11]). The isotropy at the decoupling time,t dec,at which matter and radiation decoupled,universe was very homogeneous, as showed by the simple relation:δρT(28)([13])4.The difference between the actual universe and that at decoupling is evident.The transformation between a highly homogeneous universe,at early times,to an highly local non homogeneous one,can be explained supposing that at t dec were present small inhomogeneities which grow up because of the gravitational instability mechanism([37]). Events leading to structure formation can be enumerated as follows:(a)Origin of quantumfluctuations at Planck epoch.(b)Fluctuations enter the horizon and they grow linearly till recombination.(c)Perturbations grow up in a different way for HDM and CDM in the post-recombination phase,till they reach the non-linear phase.(d)Collapse and structure formation.Before t dec inhomogeneities in baryonic components could not grow because photons and baryons were strictly cou-pled.This problem was not present for the CDM compo-nent.Then CDM perturbations started to grow up before those in the baryonic component when universe was mat-ter dominated.The epoch t eq≈4.4∗1010(Ω0h2)−2sec,at which matter and radiation density are almost equal,can be considered as the epoch at which structures started to form.The study of structure formation is fundamentally an initial value problem.Data necessary for starting this study are:1)Value ofΩ0.In CDM models the value chosen for this parameter is1,in conformity with inflationary theory pre-dictions.2)The values ofΩi for the different components in the uni-verse.For example in the case of baryons,nucleosynthesis gives us the limit0.014≤Ωb≤0.15whileΩW IMP S≈0.9.3)The perturbation spectrum and the nature of pertur-bations(adiabatic or isocurvature).The spectrum gener-ally used is that of Harrison-Zeldovich:P(k)=Ak n with n=1.The perturbation more used are adiabatic or curva-ture.This choice is dictated from the comparison between theory and observations of CMBR anisotropy.1.4The spectrum of density perturbationIn order to study the distribution of matter density in the universe it is generally assumed that this distribution is given by the superposition of plane waves independently evolving,at least until they are in the linear regime(this means till the overdensityδ=ρ−ρthe average density in the volume and withρ(r)the density in r,it is possible to define the density contrast as:δ(r)=ρ(r)−l(and similar conditions for the other components)and for the periodicity conditionδ(x,y,L)=δ(x,y,0)(and similar conditions for the other components). Fourier coefficientsδk are complex quantities given by:δk=1σ2)(32)([28]).The quantityσthat is present in Eq.(32)is the variance of the densityfield and is defined as:σ2=<δ2>= k<|δk|2>=1(2π)3 P(k)d3k=17It can be defined as the joint probability of finding an over-density δin two distinct points of space:ξ(r ,t )=<δ(r ,t )δ(r +x ,t )>(35)([38]),where averages are averageson anensemble obtainedfromseveralrealizations ofuniverse.Correlation functioncan be expressed as the joint probability of finding a galaxy in a volume δV 1and another in a volume δV 2separated by a distance r 12:δ2P =n 2V [1+ξ(r 12)]δV 1δV 2(36)where n V is the average number of galaxies per unit volume.The concept of correlation function,given in this terms,can be enlarged to the case of three or more points.Correlation functions have a fundamental role in the study of clustering of matter.If we want to use this function for a complete description of clustering,one needs to know the correlation functions of order larger than two ([39]).By means of correlation functions it is possible to study the evolution of clustering.The correlation functions are,in fact,connected one another by means of an infinite system of equations obtained from moments of Boltzmann equa-tion which constitutes the BBGKY (Bogolyubov-Green-Kirkwood-Yvon)hierarchy ([40]).This hierarchy can be transformed into a closed system of equation using closure conditions.Solving the system one gets information on cor-relation functions.In order to show the relation between perturbation spec-trum and two-points correlation function,we introduce inEq.(35),Eq.(30),recalling that δ∗k =δ(−k )and taking the limit V u →∞,the average in the Eq.(35)can be expressed in terms of the integral:ξ(r )=12π2k 2P (k )sin (kr )b (t p )2T 2(k ;t f )P (k ;t p )(39)where b(t)is the law of grow of perturbations,in the linear regime.In the case of CDM models the transfer function is:T (k )= 1+ ak +(bk )1.5+(ck )2 ν−1S=3ρr−δρmT−δρm8The distribution function f that appears in the previous equations cannot be obtained from observations.It is possi-ble to measure moments of f (density,average velocity,etc.).We want now to obtain the evolution equations for δ.Forthis reason,we start integrating Eq.(44)on p and after using Eq.(43),we get:a 3ρb∂δa 2▽p fd 3p =0(45)If we define velocity as:v =pfd 3p(46)and introduce it in Eq.(45)we get:ρb ∂δa▽(ρv )=0(47)We can now multiply Eq.(44)for p and integrate it on the momentum:∂ma 2∂βp αp βfd 3p +a 3ρ(x ,t )φ,α=0(48)this last in Eq.(45)leaves us with:∂2δa ∂δa2▽[(1+δ)▽φ]+1ma 2fd 3p(50)the equation for the evolution of overdensity becomes:∂2δa ∂δa 2▽[(1+δ)▽φ]+1∂t 2+2˙a ∂t=4πGρb δ(52)This equation in an Einstein-de Sitter universe (Ω=1,Λ=0)has the solutions:δ+=A +(x )t2a 2=82(1−Ω0)(cosh η−1)(55)t (η)=Ω0a 2=823t.(57)Before concluding this section,we want to find an ex-pression for the velocity field in the linear ingthe equation of motion p =ma 2˙x ,d pdt+v ˙a a=Gρb ad 3xδ(x ,t )x −x ′4π∂|x ′−x |(59)([38]).This solution is valid just as that for δin the linear regime.At time t =t 0this regime is valid on scales larger than 8h −1Mpc .1.7Non-linear phaseLinear evolution is valid only if δ<<1or similarly,if the mass variance,σ,is much less than unity.When this condi-tion is no longer verified (e.g.,if we consider scales smaller than 8h −1Mpc),it is necessary to develope a non-linear theory.In regions smaller than 8h −1Mpc galaxies are not a Poisson distribution but they tend to cluster.If one wants to study the properties of galactic structures or clusters of galaxies,it is necessary to introduce a non-linear theory of clustering.A theory of this last item is too complicated to be developed in a purely theoretical fashion.The problem can be faced assuming certain approximations that simpli-fies it ([47])or as often it is done,by using N-Body simu-lations of the interesting system.The approximations are often used to furnish the initial data to simulations.In the simulations,a large number of particles are randomly dis-tributed in a sphere,in the points of a cubic grid,in order to eliminate small scale noise.The initial spectrum is ob-tained perturbing the initial positions by means of a super-position of plane waves having random distributed phases and wave vector ([48]).Obviously,the universe is considered in expansion (or comoving coordinates are used),and then the equation of motion of particles are numerically solved.For what concerns the analytical approximations one of the most used is that of [47].This gives a solution to the prob-lem of the grow of perturbations in an universe with p =0not only in the linear regime but even in the mildly non-linear regime.In this approximation,one supposes to have particles with initial position given in Lagrangian coordi-nates q .The positions of particles,at a given time t,are given by:9 where x indicates the Eulerian coordinates,p(q)describesthe initial densityfluctuations and b(t)describes their growin the linear phase and it satisfies the equation:d2bdtdadt =dbρ ∂q jρ δjk+b(t)∂p k3p(q)= k i k3exp(i kq)(67)([28]),that leads us back to the linear theory.In other words,Ze‘ldovich approximation is able to reproduce the linear theory,and is also able to give a good approximationin regions withδρρ>>ing the expression for p(q),theJacobian in Eq.(64)is a real matrix and symmetric that can be diagonalized.With this p(q)the perturbed density can be written as:ρ(q,t)=(1−b(t)λ1(q))(1−b(t)λ2(q))(1−b(t)λ3(q))(68) whereλ1,λ2,λ3are the three eigenvalues of the Jacobian, describing the expansion and contraction of mass along the principal axes.From the structure of the last equation,we notice that in regions of high density Eq.(68)becomes infinite and the structure of collapse in a pancake,in a filamentary structure or in a node,according to values of eigenvalues.Some N-body simulations([49])tried to ver-ify the prediction of Ze‘ldovich approximation,using initial conditions generated using a spectrum with a cut-offat low frequencies.The results showed a good agreement between theory and simulations,for the initial phases of the evolu-tion(a(t)=3.6).Going on,the approximation is no more valid starting from the time of shell-crossing.After shell-crossing,particles does not oscillate any longer around the structure but they pass through it making it vanish.This problem has been partly solved supposing that particles, before reaching the singularity they sticks the one on the other,due to a dissipative term that simulates gravity and then collects on the forming structure.This model is known as“adesion-model”([50]).Summarizing,Zel’dovich approximation gives a description of the transition between linear and non-linear phase.It 1.8Quasi-linear regimeWe have seen in the previous section that in the case of regions of dimension smaller than8h−1Mpc,the linear theory is no more a good approximation and a new theory is needed or N-body simulations.Non-linear theory is able to calculate quantities as the formation redshift of a given class of objects as galaxies and clusters,the number of bound objects having masses larger than a given one,the average virial velocity and the correlation function.It is possible to get an estimate of the given quantities as that of other not cited,using an intermediate theory between the linear and non-linear theory:the quasi-linear theory. This last is obtained adding to the linear theory a model of gravitational collapse,just as the spherical collapse model.Important results that the theory gives is the bottom-up formation of structures(in the CDM model). Other important results are obtained if we identify density peaks in linear regime with sites of structure formation. Two important papers in the development of this theory are[51]and that of[52].This last paper is an application of the ideas of the quasi-linear theory to the CDM model. The principles of this approach are the following:•Regions of mass larger than M that collapsed can be identified with regions where the density contrast evolved according to linear regime,δ(M,x),has a value larger than a threshold,δc.•After collapse regions does not fragment.The major drawbacks of the theory,as described in[52]are fundamentally the fact that the estimates that can be ob-tained by means of this theory depends on the threshold δc,on the ratio between thefiltering mass and that of ob-jects and from other parameters.Nevertheless,this theory has helped cosmologists in obtaining estimate of important quantities as those previously quoted,and at same time give evidences that leads to exclude very low values for spectrum normalization.1.9Spherical CollapseSpherical symmetry is one of the few cases in which grav-itational collapse can be solved exactly([53];[38]).In fact, as a consequence of Birkhoff’s theorem,a spherical pertur-bation evolves as a FRW Universe with density equal to the mean density inside the perturbation.The simplest spherical perturbation is the top-hat one, i.e.a constant overdensityδinside a sphere of radius R; to avoid a feedback reaction on the background model,the overdensity has to be surrounded by a spherical underdense shell,such to make the total perturbation vanish.The evo-lution of the radius of the perturbation is then given by a Friedmann equation.The evolution of a spherical perturbation depends only on its initial overdensity.In an Einstein-de Sitter background, any spherical overdensity reaches a singularity(collapse)at afinal time:t c=3π3δ(t i) −3/2t i.(69) By that time its linear density contrast reaches the value:3/2。
Investigating the mysteries of dark matter

Investigating the mysteries of darkmatterThe universe can be a mysterious place, and one of the biggest mysteries at the moment is that of dark matter. Dark matter accounts for approximately 85% of the matter in the universe, but we still don't know what it's made of. There are many theories and hypotheses, but nothing has been proven yet. So, what is dark matter, and why is it so important?Observations and Galactic Rotation CurvesThe existence of dark matter was first suggested in the 1930s when Swiss astronomer Fritz Zwicky noticed that the mass of galaxy clusters seemed to be much more than the mass of visible matter. The idea was largely ignored until the 1970s, when Vera Rubin and Kent Ford noticed that the rotation curves of spiral galaxies also implied the presence of more mass than was observable. Essentially, these galaxies were rotating too fast for the amount of visible matter to hold them together. From these observations, it was concluded that there had to be some other form of matter, invisible and undetectable, that was influencing the motion of the galaxies.Dark Matter CandidatesThere are many possible explanations for dark matter, but scientists have yet to identify a definitive candidate. One of the most popular theories is that dark matter is made up of weakly interacting massive particles (WIMPs). These particles would be extremely difficult to detect, but efforts have been made to find them through instruments such as the Large Hadron Collider and the Dark Energy Survey.Other possible candidates for dark matter include axions, sterile neutrinos, and primordial black holes. Despite decades of research, none of these theories has yet been proven to be the source of dark matter.The Importance of Dark MatterSo why is dark matter important? In addition to accounting for most of the matter in the universe, it also plays a crucial role in the formation of galaxy clusters and large-scale structures in the universe. Without dark matter, the early universe would have been unable to form the structures we see today. Dark matter also affects the cosmos on a more fundamental level, influencing the distribution of light and matter across the universe.ConclusionDark matter is one of the most intriguing mysteries in the universe, but we are getting closer to understanding it. As technology advances, we may be able to detect the particles that make up dark matter and finally unravel the mystery. Until then, scientists will continue to theorize and experiment, searching for answers to one of the biggest questions in cosmology.。
The Direct Detection of Non-Baryonic Dark Matter in the Galaxy

遵义“PEP”2024年小学6年级B卷英语第六单元自测题
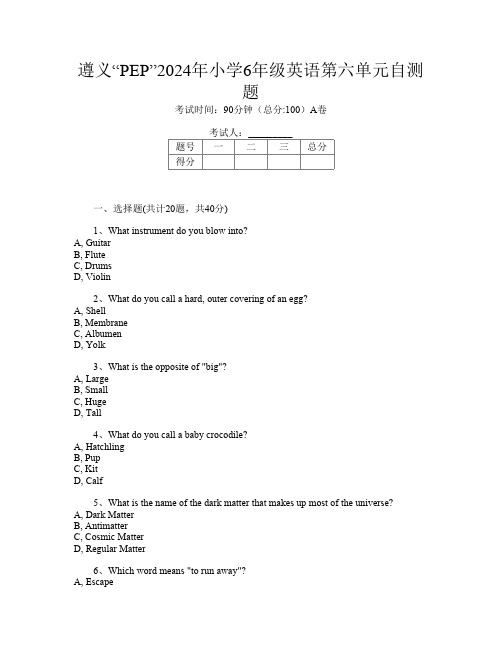
遵义“PEP”2024年小学6年级英语第六单元自测题考试时间:90分钟(总分:100)A卷考试人:_________题号一二三总分得分一、选择题(共计20题,共40分)1、What instrument do you blow into?A, GuitarB, FluteC, DrumsD, Violin2、What do you call a hard, outer covering of an egg?A, ShellB, MembraneC, AlbumenD, Yolk3、What is the opposite of "big"?A, LargeB, SmallC, HugeD, Tall4、What do you call a baby crocodile?A, HatchlingB, PupC, KitD, Calf5、What is the name of the dark matter that makes up most of the universe?A, Dark MatterB, AntimatterC, Cosmic MatterD, Regular Matter6、Which word means "to run away"?A, EscapeB, ArriveC, StayD, Return7、Which food is typically eaten at breakfast?A, PizzaB, CerealC, HamburgerD, Pasta8、What is the primary color of a cantaloupe melon?A, OrangeB, GreenC, YellowD, Red9、Which planet is known for its rings?A, JupiterB, SaturnC, NeptuneD, Uranus10、How many vowels are in the English alphabet?A, FiveB, SixC, SevenD, Four11、What is the name of the fairy tale character with long hair?A, CinderellaB, RapunzelC, Snow WhiteD, Beauty12、Which animal is known as man's best friend?A, CatB, DogC, RabbitD, Parrot13、What do you call the time it takes for the Earth to go around the Sun? A, MonthB, YearC, WeekD, Day14、What color is the sky on a clear day?A, GreenB, BlueC, RedD, Yellow15、What is the name of the first woman to fly in space?A, Sally RideB, Valentina TereshkovaC, Mae JemisonD, Eileen Collins16、Where do fish live?A, TreesB, WaterC, LandD, Sky17、What do we call the circular path a celestial object takes as it revolves around another?A, OrbitB, TrajectoryC, PathwayD, Route18、What do you call a collection of maps?A, AtlasB, DictionaryC, EncyclopediaD, Almanac19、What do we call a baby sheep?A, CalfB, LambC, KidD, Foal20、What do we call the study of living things?A, ChemistryB, BiologyC, PhysicsD, Geography二、听力题(共计20题,共40分)I want to ___ an astronaut. (become)2、听力题:The monkey is _____ (swinging/sitting) in the tree.3、听力题:The chemical formula for glucose is ______.4、听力题:The capital of San Marino is __________.5、听力题:Metals are usually ______ at room temperature.6、听力题:Planets can be _____ or gas giants.7、听力题:The chemical bond formed by sharing electrons is called a __________ bond.8、听力题:The trees are ___ (swaying) in the wind.9、听力题:To neutralize an acid, you can add a ______.10、听力题:The acidity of a solution can be measured using a ______.11、听力题:In chemistry, a compound is made of two or more different ______.12、听力题:Many _______ have interesting facts and histories.13、听力题:__________ energy is required to break chemical bonds.14、听力题:Elements are organized in the _______ table.15、听力题:A __________ is an important resource for agriculture.My grandmother tells the best ____ (stories) before bed.17、听力题:A solution with a lower concentration of solute is said to be ______.18、听力题:In a double displacement reaction, two compounds exchange _____ to form new compounds.19、听力题:Space probes collect data from distant ______.20、听力题:The chemical symbol for chlorine is ______.三、填空题(共计20题,共10分)1、填空题:The __________ (历史的启发) can ignite passion.2、填空题:I found a _____ (蘑菇) in the forest.3、填空题:The __________ (历史的剪影) captures essence.4、填空题:A ___ (小蝙蝠) flies at dusk.5、填空题:My favorite animal is the _____ (狗).6、填空题:_____ (草本植物) are used in many recipes.7、填空题:I love my _____ (毛绒玩具) for cuddles.8、填空题:The _______ (鸡) lays eggs.The __________ (历史的叙述者) play crucial roles in shaping narratives.10、填空题:A _____ (leaf) changes color in autumn.11、填空题:A _____ (海豹) basks in the sun on the rocks.12、填空题:The __________ (历史的理解) requires critical thinking.13、填空题:The tree gives us _______ (这棵树给我们_______).14、填空题:My ________ (玩具名称) has a treasure map.15、填空题:The capital of Denmark is ________ (哥本哈根).16、填空题:A _____ (植物专家) gives advice on caring for plants.17、填空题:The tortoise is known for its hard ________________ (壳).18、填空题:The __________ (分子量) is the sum of the atomic weights of all atoms in a molecule.19、填空题:The _____ (树) is tall and strong.20、填空题:I have a collection of ______ (硬币) from around the world. They are very ______ (特别).。
基于伽马射线的类轴子粒子探测及暗物质子晕搜寻研究
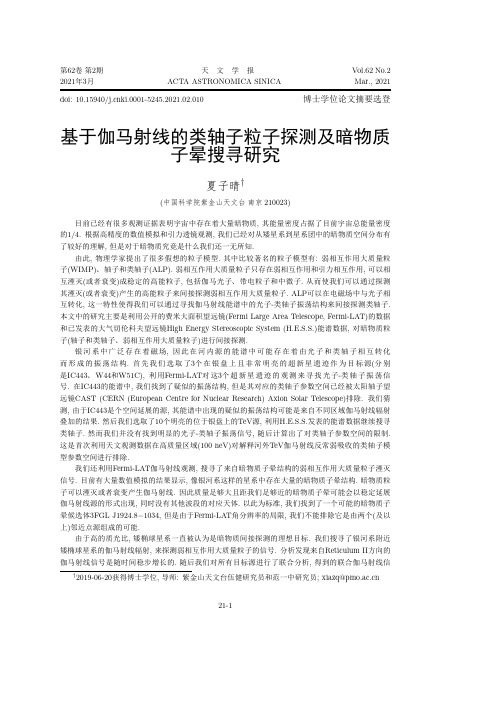
第62卷第2期天文学报Vol.62No.2 2021年3月ACTA ASTRONOMICA SINICA Mar.,2021doi:10.15940/ki.0001-5245.2021.02.010博士学位论文摘要选登基于伽马射线的类轴子粒子探测及暗物质子晕搜寻研究夏子晴†(中国科学院紫金山天文台南京210023)目前已经有很多观测证据表明宇宙中存在着大量暗物质,其能量密度占据了目前宇宙总能量密度的1/4.根据高精度的数值模拟和引力透镜观测,我们已经对从矮星系到星系团中的暗物质空间分布有了较好的理解,但是对于暗物质究竟是什么我们还一无所知.由此,物理学家提出了很多假想的粒子模型.其中比较著名的粒子模型有:弱相互作用大质量粒子(WIMP)、轴子和类轴子(ALP).弱相互作用大质量粒子只存在弱相互作用和引力相互作用,可以相互湮灭(或者衰变)成稳定的高能粒子,包括伽马光子、带电粒子和中微子.从而使我们可以通过探测其湮灭(或者衰变)产生的高能粒子来间接探测弱相互作用大质量粒子.ALP可以在电磁场中与光子相互转化,这一特性使得我们可以通过寻找伽马射线能谱中的光子-类轴子振荡结构来间接探测类轴子.本文中的研究主要是利用公开的费米大面积望远镜(Fermi Large Area Telescope,Fermi-LAT)的数据和已发表的大气切伦科夫望远镜High Energy Stereoscopic System(H.E.S.S.)能谱数据,对暗物质粒子(轴子和类轴子、弱相互作用大质量粒子)进行间接探测.银河系中广泛存在着磁场,因此在河内源的能谱中可能存在着由光子和类轴子相互转化而形成的振荡结构.首先我们选取了3个在银盘上且非常明亮的超新星遗迹作为目标源(分别是IC443、W44和W51C),利用Fermi-LAT对这3个超新星遗迹的观测来寻找光子-类轴子振荡信号.在IC443的能谱中,我们找到了疑似的振荡结构,但是其对应的类轴子参数空间已经被太阳轴子望远镜CAST(CERN(European Centre for Nuclear Research)Axion Solar Telescope)排除.我们猜测,由于IC443是个空间延展的源,其能谱中出现的疑似的振荡结构可能是来自不同区域伽马射线辐射叠加的结果.然后我们选取了10个明亮的位于银盘上的TeV源,利用H.E.S.S.发表的能谱数据继续搜寻类轴子.然而我们并没有找到明显的光子-类轴子振荡信号,随后计算出了对类轴子参数空间的限制.这是首次利用天文观测数据在高质量区域(100neV)对解释河外TeV伽马射线反常弱吸收的类轴子模型参数空间进行排除.我们还利用Fermi-LAT伽马射线观测,搜寻了来自暗物质子晕结构的弱相互作用大质量粒子湮灭信号.目前有大量数值模拟的结果显示,像银河系这样的星系中存在大量的暗物质子晕结构.暗物质粒子可以湮灭或者衰变产生伽马射线.因此质量足够大且距我们足够近的暗物质子晕可能会以稳定延展伽马射线源的形式出现,同时没有其他波段的对应天体.以此为标准,我们找到了一个可能的暗物质子晕候选体3FGL J1924.8−1034,但是由于Fermi-LAT角分辨率的局限,我们不能排除它是由两个(及以上)邻近点源组成的可能.由于高的质光比,矮椭球星系一直被认为是暗物质间接探测的理想目标.我们搜寻了银河系附近矮椭球星系的伽马射线辐射,来探测弱相互作用大质量粒子的信号.分析发现来自Reticulum II方向的伽马射线信号是随时间稳步增长的.随后我们对所有目标源进行了联合分析,得到的联合伽马射线信†2019-06-20获得博士学位,导师:紫金山天文台伍健研究员和范一中研究员;21-12天文学报62卷号已经超过了4σ的局域置信度.在暗物质间接探测中,主要困难在于如何把暗物质湮灭或衰变产物的信号从天体物理背景中分离出来.如果是搜寻具有某些独特特征的能谱,如线谱和箱型能谱,在这方面遇到的困难就要小一些,因为通常的天体物理辐射过程难以出现这种特殊结构的能谱.在本文的工作中,我们还利用了Fermi-LAT数据来搜寻暗物质粒子可能产生的特征能谱(包括线谱和箱型能谱)信号.我们分别在银河系卫星星系和银河系内的暗物质子晕结构(通过N体模拟)寻找潜在的线谱信号.由于没有发现明显信号,我们随后计算出了暗物质湮灭成两个光子的湮灭截面的相应上限.随后我们还在矮椭球星系中,研究了由暗物质湮灭或衰变所产生的中间粒子衰变发出的箱型伽马射线能谱信号.Probe Axion-like Particles(ALPs)and Search for Dark Matter Subhalo with the Gamma-rayObservationsXIA Zi-qing(Purple Mountain Observatory,Chinese Academy of Sciences,Nanjing210023)The presence of a large amount of dark matter(DM)in the Universe has already been convincingly established.DM is believed to make up a quarter of the energy density of the current Universe.Thanks to high-resolution numerical simulations made possible by modern supercomputers and the gravitational lensing observations,the distribution of DM in structures ranging from dwarf galaxies to clusters of galaxies has been understood better than before.But the nature of DM remains unknown.Various hypothetical particles have been proposed,such as weakly-interacting mas-sive particles(WIMPs),axion,axion-like particles(ALPs),sterile neutrino and gravitino. WIMPs may be able to annihilate with each other(or alternatively decay)into stable high-energy particle pairs,including gamma-rays,charged particles and neutrinos.ALPs and photons can convert to each other in electromagneticfields through the Primakoffprocess, which could result in the detectable spectral oscillation phenomena in the gamma-rays ob-servation.My research mainly focused on the indirect detection of dark matter,such as ALPs and WIMPs,using publicly available Fermi Large Area Telescope(Fermi-LAT)data and the the published data of High Energy Stereoscopic System(H.E.S.S.)observation.The conversion between photons and ALPs in the Milky Way magneticfield could result in the detectable oscillation phenomena in the gamma-ray spectra of the Galactic sources. First,we search for such oscillation effects in the spectra of supernova remnants caused by the photon-ALP conversion,using the Fermi LAT data.The inclusion of photon-ALP oscillations yields an improvedfit to theγ-ray spectrum of IC443,which gives a statistical significance of4.2σin favor of such spectral oscillation.However,the best-fit parameters of ALPs are in tension with the CAST(CERN(European Centre for Nuclear Research)Axion Solar Telescope)limits.Secondly,we use the H.E.S.S.observations of some TeV sources in the Galactic plane to exclude the highest ALP mass region(i.e.,ALP mass m a∼10−7eV) that accounts for the anomalously weak absorption of TeV gamma-rays for thefirst time.A Milky Way-like galaxy is predicted to host tens of thousands of galactic DM subhalos. Annihilation of WIMPs in massive and nearby subhalos could generate detectable gamma-rays,appearing as unidentified,spatially-extended and stable gamma-ray sources.We search for such sources in the third Fermi Large Area Telescope source List(3FGL)and report21-22期夏子晴:基于伽马射线的类轴子粒子探测及暗物质子晕搜寻研究3the identification of a new candidate,3FGL J1924.8−1034.3FGL J1924.8−1034is found spatially-extended at a high confidence level of5.4σ.No significant variability has been found and its gamma-ray spectrum is wellfitted by the dark matter annihilation into b¯b with a mass of∼43GeV.All these facts make3FGL J1924.8−1034a possible dark matter subhalo candidate.However,due to the limited angular resolution,the possibility that the spatial extension of3FGL J1924.8−1034is caused by the contamination from the other un-resolved point source can not be ruled out.The Milky Way dwarf spheroidal galaxy is considered one of the most ideal targets for indirect detection of dark matter due to their high dark matter density and low astrophysical backgrounds.We search for gamma-ray emission from nearby Milky Way dwarf spheroidal galaxies and candidates with Fermi-LAT data.Intriguingly,the peak TS(Test Statistic) value of the weak emission from Reticulum II rises continually.We alsofind that the combination of all these nearby sources will result in a more significant(>4σ)gamma-ray signal.A commonly encountered obstacle in indirect searches for dark matter is how to disentangle possible signals from astrophysical backgrounds.Gamma-ray features,in particular monochromatic gamma-ray lines and boxlike spectral features,provide smoking gun signatures.We analyze the Fermi LAT observation of Milky Way satellites and the local volume dark matter subhalo population(with N-body simulation)to search for potential line signals,respectively.The corresponding upper limits on the cross section of DM annihilation into two photons are derived,without significant signal found.Then we study the box-shaped DM signals,which is generated by the decay of intermediate particles produced by DM annihilation or decay,with Fermi-LAT observations of dwarf spheroidal galaxies.21-3。
介绍未解之谜的英语作文
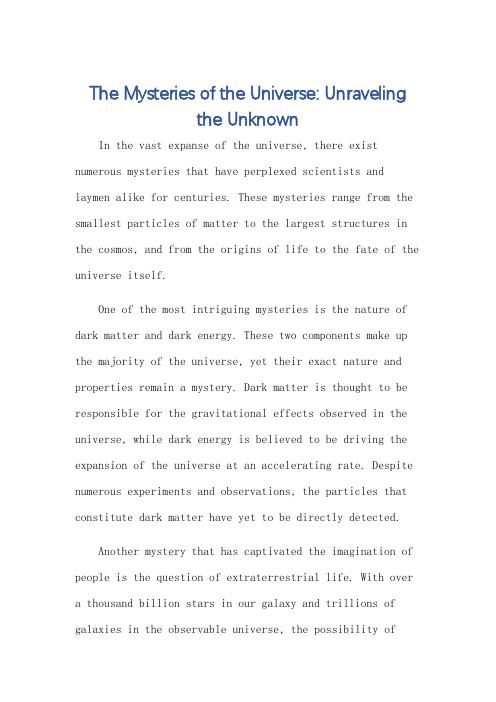
The Mysteries of the Universe: Unravelingthe UnknownIn the vast expanse of the universe, there exist numerous mysteries that have perplexed scientists and laymen alike for centuries. These mysteries range from the smallest particles of matter to the largest structures in the cosmos, and from the origins of life to the fate of the universe itself.One of the most intriguing mysteries is the nature of dark matter and dark energy. These two components make up the majority of the universe, yet their exact nature and properties remain a mystery. Dark matter is thought to be responsible for the gravitational effects observed in the universe, while dark energy is believed to be driving the expansion of the universe at an accelerating rate. Despite numerous experiments and observations, the particles that constitute dark matter have yet to be directly detected.Another mystery that has captivated the imagination of people is the question of extraterrestrial life. With over a thousand billion stars in our galaxy and trillions of galaxies in the observable universe, the possibility oflife existing elsewhere seems inevitable. However, despite searches that span the electromagnetic spectrum and include missions to Mars and beyond, no conclusive evidence of extraterrestrial life has been found.The origin of life on Earth is also a mystery that remains unsolved. How did life arise from non-living matter? How did the first cells form and evolve into the diverse array of organisms we see today? These questions have been studied by biologists, biochemists, and physicists for centuries, but the answers remain elusive.Quantum mechanics, a branch of physics that deals with the behavior of matter and energy at the atomic and subatomic levels, is also full of mysteries. Phenomena such as entanglement, superposition, and tunneling defy common sense and intuition. These quantum effects have been observed and verified through numerous experiments, buttheir interpretation and understanding remain a challenge.The mysteries of the universe are not just scientific curiosities; they are fundamental questions about our existence and the nature of reality. As science continuesto advance, we may unravel some of these mysteries and gaina deeper understanding of the universe. However, there will always be new mysteries to explore and new questions to ask. The journey of discovery is an ongoing one, and it is this quest for knowledge that drives us forward.**宇宙之谜:揭开未知的面纱**在浩瀚无垠的宇宙中,存在着许多数百年来一直困扰着科学家和普通人的未解之谜。
- 1、下载文档前请自行甄别文档内容的完整性,平台不提供额外的编辑、内容补充、找答案等附加服务。
- 2、"仅部分预览"的文档,不可在线预览部分如存在完整性等问题,可反馈申请退款(可完整预览的文档不适用该条件!)。
- 3、如文档侵犯您的权益,请联系客服反馈,我们会尽快为您处理(人工客服工作时间:9:00-18:30)。
a r X i v :h e p -p h /0107151v 2 15 J u l 2001ACT-07/01,CTP-TAMU-24/01CERN-TH/2001-168,UA/NPPS-8-01HEPHY-PUB 741/01hep-ph/0107151Dark Matter Direct Searches and the Anomalous Magnetic Moment of Muon hanas 1, D.V.Nanopoulos 2and V.C.Spanos 31University of Athens,Physics Department,Nuclear and Particle Physics Section,GR–15771Athens,Greece 2Department of Physics,Texas A &M University,College Station,TX 77843-4242,USA,Astroparticle Physics Group,Houston Advanced Research Center (HARC),Mitchell Campus,Woodlands,TX 77381,USA,and Academy of Athens,Chair of Theoretical Physics,Division of Natural Sciences,28Panepistimiou Avenue,Athens 10679,Greece 3Institut f¨u r Hochenergiephysik der ¨Osterreichischen Akademie der Wissenschaften,A–1050Vienna,Austria AbstractIn the framework of the Constrained Minimal Supersymmetric Standard Model(CMSSM)we discuss the impact of the recent experimental information,especially from E821Brookhaven experiment on g µ−2along with the light Higgs boson mass bound from LEP,to the Dark Matter direct searches.Imposing these experimen-tal bounds,the maximum value of the spin-independent neutralino-nucleon cross section turns out to be of the order of 10−8pb for large values of tan βand low M 1/2,m 0.The effect of the recent experimental bounds is to decrease the maxi-mum value of the cross section by about an order of magnitude,demanding the analogous sensitivity from the direct Dark Matter detection experiments.Supersymmetry,or fermion-boson symmetry,is an omnipotent and ubiquitous ele-ment in our efforts to construct a unified theory of all fundamental interactions observed in nature.At very high energies,close to the Planck scale(M P)it is indispensable in con-structing consistent string theories,thus dubbed superstrings.At low energies(∼1TeV) it seems unavoidable if the gauge hierarchy problem is to be resolved.Such a resolution provides a measure of the supersymmetry breaking scale M SUSY≈O(1TeV).There is, albeit circumstantial or indirect,evidence for such a low-energy supersymmetry breaking scale,from the unification of the gauge couplings[1]and from the apparent lightness of the Higgs boson as determined from precise electroweak measurements,mainly at LEP[2].Furthermore,such a low energy SUSY breaking scale is also favored cosmo-logically.As is well known,R-parity conserving SUSY models,contain in the sparticle spectrum a stable,neutral particle,identifiable with the lightest neutralino(˜χ),referred as the LSP[3].One can then readily show[3]that such a LSP with mass,as low-energy SUSY entails,in the100GeV−1TeV region,may indeed provide the right form and amount of the highly desirable astrophysically and cosmologically Dark Matter(DM). As times goes by,the experimental evidence for DM,from different quarters,strengthens in such a way,that it has assumed a central role in the modern cosmology.The most recent evidence,coming from the observation of thefirst three acoustic peaks in the Cos-mic Microwave Background(CMB)radiation small angle(θ O(10)anisotropies[4], is of tantalizing importance.It is not only provides strong support to aflat(k=0 orΩ0=1),inflationary Universe,but it also gives an unprecedented determination of ΩM h20≈0.15±0.05,which taking into account the simultaneously determined baryon densityΩB h20≈0.02,and the rather minute neutrino density suggestsΩDM h20=0.13±0.05(1)One then is tempted to combine this recently determined DM density,assuming,as we do here,that it is all due to neutralini(i.e.ΩDM≡Ω˜χ),with other presentlyavailable constraints from particle physics,in order tofind out what is the chances ofobserving,soon or in the near future,DM directly in the laboratory by elastic neutralino-nucleus scattering,from the energy deposition in the detectors[5].These particle physicsconstraints include the lower bound on the mass of the Higgs bosons(m h≥113.5GeV) provided by LEP[6],the allowed region for b→sγ,at95%CL range(2.33×10−4<B(b→sγ)<4.45×10−4)[7],and the recent results from the BNL E821experiment[8] on the anomalous magnetic moment of the muon(δαµ=43(16)×10−10),assuming,as we do here,that is all due(at the1or2σlevel)to low-energy supersymmetry.It should be stressed that the possibility of a rather sizeable positive contribution to gµ−2from low energy SUSY in the region of large tanβandµ>0where the b→sγconstraint weakens considerably,has been long strongly emphasized[9].It is amusing to notice, that in our previous analysis of the direct DM searches[10],done before the the BNL E821announcement,we had paid particular interest in the large tanβregion,since it provided the higher possible rates for direct DM detection!Similar results are presented in Ref.[11].Actually,as we have stressed for some time now[10,12],one way to get the “right”amount of the neutralino mass density(Ω˜χh20),even for relative high values of m0 and M1/2,is to move to the large tanβregion,because efficient neutralino annihilation directly through A and H poles,occurs.The annihilation cross sections increase with tanβ:couplings A˜χ˜χand Aτ¯τ,Ab¯b increase,while m A decreases,thus one may also expect a rather appreciable increase in the elastic˜χ-nucleon cross section,as is indeed the case.It may turn out,if the BNL E821result is due to low energy SUSY,that the imposed lower bounds on m0,M1/2and lower bounds on tanβ[13–16]make the direct neutralino annihilation,through the A,H poles,the major mechanism for getting the right amount of DM[10,12,17],as well as as being consistent with all available constraints[13,16].Before presenting our results we shall give a brief account on the numerical analysis employed in this paper.This will be useful in comparing our results with those of other authors.In our analysis we use two-loop renormalization group equations(RGE),in theMS strong coupling constant at M Z,which we consider as input,is related toˆα3throughαs(M Z)=ˆα3(M Z)/(1−∆ˆα3).∆ˆα3represent the threshold corrections which affect significantly the value ofˆα3at M Z and hence,through RGE,its value at M GUT.The latter turns out to be different fromˆα1,2(M GUT),reflecting the fact that gauge coupling unification is impossible to implement in the constrained scenario with universal boundary conditionsfor the soft masses.For the determination of the gauge couplingsˆα1,2we use as inputs the electromagnetic coupling constant a0the value of the Fermi coupling constant G F, and the Z-boson mass M Z.From these we determine the weak mixing angle,through √ˆs2ˆc2=πα0/DR scheme the latter is related to a0throughˆα(M Z)=a0/(1−∆ˆαem),where∆ˆαem are the appropriate threshold corrections(see Ref.[18]).The input value of the strong coupling constant is taken within the experimental rangeαs(M Z)=0.1185±0.002.In running the RGE’s,as arbitrary parameters we take are as usual the soft SUSY breaking parameters m0,M1/2,A0the value of tanβand the sign of the Higgsino mixing parameterµ.The top and tau physical masses,M t,Mτ,as well as theMS RGE’s,using three-loop RGE’s for the strong coupling constant.We also include two-loop QED corrections,as well as two-loop contributions from the interference of the QCD and QED corrections.The runningDR in the usual way.From these we can extract the corresponding Yukawa couplings at M Z.We point out that the important QCD as well as the supersymmetric gluino,sbottom and chargino,stop corrections to the bottom mass are duly taken into account.For the determination of the top Yukawa coupling at M t we relate its pole and running masses taking into account all dominant radiative corrections.By running the RGE’s we can have the value of the top Yukawa coupling at M Z.The determination of the Higgs and Higgsino mixing parameters,m23andµ,is a more subtle issue.These are obtained by solving the minimization conditions with the one-loop corrected effective potential with all particle contributions taken into account. Since large values of tanβcause large logarithmic corrections,invalidating perturbation expansion,we solve the minimization equations taking as reference scale the average stop scale Q˜t≃√m23(M Z),µ(M Z),whenever needed,can be found by solving the RGE’s having as initial conditions the values of these quantities at Q˜t.For the calculation of the lightest supersymmetric particle(LSP)relic abundance,we solve the Boltzmann equation numerically using the machinery outlined in Ref.[12].In this calculation the coannihilation effects,in regions whereτR approaches in mass the LSP,which is a high purity Bino,are properly taken into account.Before embarking to analyse our numericalfindings it would be beneficial to review the physical mechanism through which the scalar,i.e.spin-independent,˜χ-nucleon cross section(σscalar)is enhanced,to levels approaching the sensitivity of ongoing experiments. Theσscalar is enhanced in the region of the parameter space where tanβis large[10] 1.The dominant contribution to this regime is the Higgs boson exchange.For given inputs m0,M1/2,A0and the sign ofµ,Higgs masses decrease as tanβincreases.Hence the contribution of Higgs bosons to neutralino–quark elastic cross section becomes more important in the large tanβregime.Such a decrease in the mass is not sufficient by itself to increaseσscalar.The major role in this increase plays the coupling of the CP-even heavy Higgs whose coupling to d-quark is proportional to cosα1Enhancement ofσscalar is also possible in the context of the so-called focus point supersymmetry scenario[19],where m0>1.5TeV,yet such large values of m0are not favourable by the recent gµ−2 data.is less suppressive,and the increase of the˜χ˜χA as well as the Ab¯b and Aτ¯τcouplings. The smallness of the LSP’s Higgsino component is compensated by the largeness of tanβyielding neutralino annihilation cross sections compatible with the recent astrophysical data.Hence there are regions in which we can obtain both low relic densities and high σscalar.Bearing all these in mind,we proceed discussing ourfindings.For our numerical analysis a large random sample of45,000points in the region of the parameter space designed by2<tanβ<55,M1/2<1.5TeV,m0<1.5TeV,|A0|<1TeV,andµ>0is used.Theµ<0case is not favored by the recent b→sγdata,as well as by the observed discrepancy of the gµ−2,if the latter is attributed to supersymmetry,and therefore we shall not discuss it in the sequel.It is also worth noticing that in theµ>0 case the constraint from b→sγdata is superseded by the m h>113.5GeV bound, in the bulk of the parameter space[13].Infigure1we plot the scalar˜χ-nucleon cross section as function of the LSP mass,m˜χ.On the top of thefigure the shaded region(in cyan colour)is excluded by the CDMS experiment[20].The DAMA sensitivity region (coloured in yellow)is also plotted[21].Pluses(+)(in blue colour)represent points=(43.0±16.0)×10−10and the which are both compatible with the E821dataαSUSYµcosmological bounds for the neutralino relic densityΩ˜χh20=0.13±0.05.Diamonds (⋄)(in green colour)are points which are cosmologically acceptable with respect to thehas been relaxed to its2σregion,namely aforesaid bounds,but the bound to theαSUSYµ11<αSUSYµ×1010<75.The crosses(×)(in red colour)represent the rest of the points of our random sample.Here the Higgs boson mass bound,m h>113.5GeV has been properly taken into account.From thisfigure it is seen that the the points which are compatible both the gµ−2E821and the cosmological data(crosses)yield cross sections of the order of10−8−10−9pb and the maximum value of the m˜χis about200GeV.If one considers the2σregion of the gµ−2bound the preferred cross sections can be as small as10−10pb and correspondingly the upper bound of m˜χis drifted up to350GeV. In the followingfigures2and3theσscalar is plotted as function of the parameters m0 and tanβrespectively.One can see that the points which conform to cosmological and 1σgµ−2experimental constraints,yield a maximum value of m0about600GeV,and for the2σcase1200GeV.The aforementioned bounds on the m˜χand m0are relatedwith the analogous bounds put on the soft parameter M1/2and m0from the gµ−2E821 data[13,14,16].Fromfigure3it is apparent that the majority of the points that are compatible with cosmological and gµ−2data are accumulated toward rather large values of tanβ, specifically tanβ>40,although there are indeed few of them with smaller values of tanβ. As it has been already pointed out in the large tanβregion we can have simultaneously cosmologically acceptable values ofΩ˜χh20and also big values for the elastic cross section˜χ-nucleon.Furthermore the gµ−2muon data prefer large values of tanβ,asαSUSYµis proportional to tanβ[9].Therefore as tanβincreases large regions of the parameter space(m0,M1/2)are compatible with the E821experimental constraints.Taking all these into account it is not surprising that the conjunction of the cosmological and gµ−2data happens for large values of the tanβand for large scalar cross section˜χ-nucleon,as it can be perceived fromfigure3.Comparingfigure1and4one can realise how gµ−2data constrain m˜χmass to be up to200GeV or350GeV for the1σor2σcase respectively.Infigure4we don’t impose the constraints stemming from gµ−2data,therefore due to the coannihilation processes the cosmologically acceptable LSP mass can be heavier than500GeV.What is also important to be noticed about the direct searches of DM is that imposing the gµ−2data the lowest allowed˜χ-nucleon cross section increased by about2orders of magnitude,from10−11pb to10−9pb.This fact is very encouraging for the future DM direct detection experiments.Figure5illustrates the significance of the Higgs boson mass bound.If one allows for values m h>100GeV many points which yielding cross sections even O(10−7)pb paringfigure1and5we observe that the recent Higgs mass bound(m h>113.5GeV)reduces the maximum value of the scalar cross section for about one order of magnitude,that is from10−7pb to10−8pb.There is direct and indirect dependence of theσscalar on m h.As m h decreases its contribution to theσscalar,being proportional to1/m h,increases leading to largerσscalar.The indirect relation of theσscalar to m h can be perceived from Eq.2.Light m h results to light m H and therefore to largeσscalar again.Concluding we have studied the impact of the recent experimental information to the DM direct searches.Especially we have considered the effect of the recently reporteddeviation of gµ−2from its SM value,as well as of the light Higgs boson mass bound from LEP experiments.The imposition of these experimental constraints results to a maximum value for the spin-independent˜χ-nucleon cross section of the order of10−8 pb for m˜χ∼100GeV as small as allowed by chargino searches,and for tanβ>45as large as possible for the Higgs states to be as light as allowed by theoretical constraints and experimental searches.As it can be seen fromfigures4and5the effect of these experimental constraints is to decrease the maximum value ofσscalar by almost one order of magnitude,and therefore to make the direct detection of the LSP on the future experiments by some means more difficult.AcknowledgementsA.B.L.acknowledges support from HPRN-CT-2000-00148and HPRN-CT-2000-00149 programmes.He also thanks the University of Athens Research Committee for partially supporting this work.D.V.N.acknowledges support by D.O.E.grant DE-FG03-95-ER-40917and thanks the CERN Theory Division for hospitality during the completion of this work.V.C.S.acknowledges support by a Marie Curie Fellowship of the EU programme IHP under contract HPMFCT-2000-00675.References[1]J.Ellis,S.Kelley and D.V.Nanopoulos,Phys.Lett.B260(1991)131;U.Amaldi,W.de Boer and H.Furstenau,Phys.Lett.B260(1991)447;C.Giunti,C.W.Kim and U.W.Lee,Mod.Phys.Lett.A6(1991)1745.[2]LEP Electroweak Working Group,http://lepewwg.web.cern.ch/LEPEWWG/Welcome.html.[3]J.Ellis,J.S.Hagelin,D.V.Nanopoulos,K.A.Olive and M.Srednicki,Nucl.Phys.B238(1984)453;H.Goldberg,Phys.Rev.Lett.50(1983)1419.[4]A.T.Lee et.al.,MAXIMA Collaboration,astro-ph/0104459;terfield et.al.,BOOMERANG Collaboration,astro-ph/0104460;N.W.Halverson et.al.,DASI Collaboration,astro-ph/0104489;P.de Bernardis et.al.,astro-ph/0105296.[5]M.W.Goodman and E.Witten,Phys.Rev.D31(1985)3059.[6]“Searches for the Neutral Higgs Bosons of the MSSM:Preliminary Com-bined Results Using LEP Data Collected at Energies up to209GeV”,The ALEPH,DELPHI,L3and OPAL coll.,and the LEP Higgs Working Group, http://lephiggs.web.cern.ch/LEPHIGGS.[7]M.S.Alam et.al.,[CLEO Collaboration],Phys.Rev.Lett.74(1995)2885as updatedin S.Ahmed et.al.,CLEO CONF99-10;BELLE Collaboration,BELLE-CONF-0003,contribution to the30th International conference on High-Energy Physics, Osaka,2000.[8]H.N.Brown et.al.,Muon g−2Collaboration,Phys.Rev.Lett.86(2001)2227.[9]J.L.Lopez,D.V.Nanopoulos and X.Weng,Phys.Rev.D49(1994)366.[10]hanas,D.V.Nanopoulos and V.C.Spanos,Mod.Phys.Lett.A16(2001)1229,hep-ph/0009065.[11]M.Drees,Y.G.Kim,T.Kobayashi and M.M.Nojiri,Phys.Rev.D63(2001)115009.[12]hanas,D.V.Nanopoulos and V.C.Spanos,Phys.Rev.D62(2000)023515.[13]J.Ellis,D.V.Nanopoulos and K.A.Olive,Phys.Lett.B508(2001)65,hep-ph/0102331.[14]L.Everett,G.L.Kane,S.Rigolin and L.Wang,Phys.Rev.Lett.86(2001)3484;J.L.Feng and K.T.Matchev,Phys.Rev.Lett.86(2001)3480;E.A.Baltz and P.Gondolo,Phys.Rev.Lett.86(2001)5004;U.Chattopadhyay and P.Nath,hep-ph/0102157;S.Komine,T.Moroi and M.Yamaguchi,Phys.Lett.B506(2001) 93;J.Hisano and K.Tobe,hep-ph/0102315;R.Arnowitt,B.Dutta,B.Hu and Y.Santoso,Phys.Lett.B505(2001)177;K.Choi,K.Hwang,S.K.Kang,K.Y.Lee and W.Y.Song,hep-ph/0103048;S.P.Martin and J.D.Wells,Phys.Rev.D64 (2001)035003;S.Komine,T.Moroi and M.Yamaguchi,Phys.Lett.B507(2001) 224;H.Baer,C.Balazs,J.Ferrandis and X.Tata,hep-ph/0103280;Y.G.Kim and M.M.Nojiri,hep-ph/0104258;V.Barger and C.Kao,hep-ph/0106189.[15]L.Roszkowski,R.R.Austri and T.Nihei,hep-ph/0106334.[16]hanas and V.C.Spanos,hep-ph/0106345.[17]M.Drees and M.M.Nojiri,Phys.Rev.D47,376(1993);H.Baer and M.Brhlik,Phys.Rev.D53(1996)597and Phys.Rev.D57(1998)567;H.Baer,M.Brhlik, M.A.Diaz,J.Ferrandis,P.Mercadante,P.Quintana and X.Tata,Phys.Rev.D63(2001)015007;J.Ellis,T.Falk,G.Ganis,K.A.Olive and M.Srednicki, hep-ph/0102098.[18]J.A.Bagger,K.Matchev,D.M.Pierce and R.-J.Zhang,Nucl.Phys.B491(1997)3.[19]J.L.Feng,K.T.Matchev and F.Wilczek,Phys.Lett.B482(2000)388.[20]R.Abusaidi et.al.,CDMS Collaboration,Phys.Rev.Lett.84(2000)5699.[21]R.Bernabei et.al.,DAMA Collaboration,Phys.Lett.B480(2000)23.Figure1:Scatter plot of the scalar neutralino-nucleon cross section versus m˜χ,from a random sample of45,000points.On the top of thefigure the CDMS excluded region and the DAMA sensitivity region are illustrated.Pluses(+)are points within the E821experimental regionαSUSYµ=(43.0±16.0)×10−10and also cosmologically acceptableΩ˜χh20=0.13±0.05.Diamonds(⋄)are also cosmologically acceptable points,but withαSUSY µwithin the region11×10−10<αSUSYµ<75×10−10.Crosses(×)represent therest of the random sample.The Higgs boson mass bound m h>113.5GeV is properly taking into account.Figure2:In thisfigure we display the scalar neutralino-nucleon cross section versus m0. Points are as in Fig.1.Figure3:In thisfigure we display the scalar neutralino-nucleon cross section versus tanβ.Points are as in Fig.1.Figure4:Scatter plot of the scalar neutralino-nucleon cross section versus m˜χ,from a random sample of Fig.1.Diamonds(⋄)are cosmologically acceptable points,withoutputting an restriction from theαSUSYµ.Crosses(×)represent points with unacceptableΩ˜χh20.Figure5:Scalar neutralino-nucleon cross section versus m˜χ.Points are as in Fig.1. Here the Higgs boson mass bound(m h>113.5GeV)has been relaxed and the bound m h>100GeV is used.。