Responsive Polymers at the Biology Materials
展望21世纪的聚合物疗法

Polymer therapeutics-prospects for 21st century:The end of the beginning ☆Ruth Duncan ⁎,1,María J.Vicent ⁎⁎Polymer Therapeutics Lab.,Centro de Investigación Príncipe Felipe,ardo Primo Yúfera 3E-46012,Valencia,Spaina b s t r a c ta r t i c l e i n f o Article history:Accepted 22August 2012Available online 5September 2012Keywords:NanomedicineBlock copolymer micelle Dendrimer Endocytosis AptamerPolymer therapeutic biomarkerThe term “polymer therapeutics ”was coined to describe polymeric drugs,polymer conjugates of proteins,drugs and aptamers,together with those block copolymer micelles and multicomponent non-viral vectors which con-tain covalent linkages.These often complex,multicomponent constructs are actually “drugs ”and “macromolec-ular prodrugs ”in contrast to drug delivery systems that simply entrap (non-covalently)therapeutic agents.They have also been described as nanomedicines.First polymer –protein conjugates entered routine clinical use in 1990and a growing number of polymeric drugs/sequestrants and PEGylated proteins/aptamers have since come into the market.Valuable lessons have been learnt over >3decades of clinical development,especially in relation to critical product attributes governing safety and ef ficacy,the validated methods needed for product characterisation.Not least there has been improved understanding of polymer therapeutic-speci fic biomarkers that will in future enable improved selection of patients for therapy.Advances in synthetic polymer chemistry (including control of 3D architecture),the move towards greater use of biodegradable polymers,polymers deliv-ering combination therapy,increased understanding of polymer therapeutic critical product attributes to guide pharmaceutical development,and advances in understanding of endocytosis and intracellular traf ficking path-ways in health and disease are opening new opportunities for design and clinical use of polymer-based therapeu-tics in the decades to come.©2012Elsevier B.V.All rights reserved.Contents 1.The beginnings .............................................................601.1.Introduction ...........................................................601.2.Evolution of polymer therapeutics .................................................612.The future ................................................................642.1.Chemistry and analytical tools ...................................................642.2.Clinical applications and polymer therapeutic biomarkers ......................................683.Conclusions ...............................................................69Acknowledgements ..............................................................69References ............................ (69)1.The beginnings 1.1.IntroductionOn the occasion of the “25th birthday ”of Advanced Drug Delivery Reviews it is timely to re flect on the progress made across the polymertherapeutics sector (Fig.1a),and consider the potential for further de-velopments into the 21st Century.The field has certainly come a long way since its beginnings,and arguably polymer therapeutics have been amongst the most successful first generation nanomedicines (reviewed in Ref.[1])considering the relatively modest investment made in these technologies (active researchers and funding)during the last quarter of the 20th Century compared to liposomes,nanoparticles,biodegradable polymeric implants and immunoconjugates and other ad-vanced drug delivery systems for oral delivery.It is truly the “end of the beginning ”!In 2013we celebrate the 60th anniversary of the 1953Hermann Staudinger Nobel Prize,the first awarded for “polymer ”chemistry.Not only did Staudinger give us the concept of covalently linked “macromolecules ”he foresaw the potential of their use inAdvanced Drug Delivery Reviews 65(2013)60–70☆This review is part of the Advanced Drug Delivery Reviews theme issue on "25th Anniversary issue -Advanced Drug Delivery:Perspectives and Prospects".⁎Corresponding author.Tel.:+442920916160.⁎⁎Corresponding author.Tel.:+34963289681x2307#.E-mail addresses:profruthduncan@ (R.Duncan),mjvicent@cipf.es (M.J.Vicent).1Ruth Duncan is Visiting Professor at CIPF,Valencia.0169-409X/$–see front matter ©2012Elsevier B.V.All rights reserved./10.1016/j.addr.2012.08.012Contents lists available at SciVerse ScienceDirectAdvanced Drug Delivery Reviewsj o u r n a l h om e p a g e :ww w.e l s e v i e r.c o m /l o c a t e /a d d rbiomedical applications [2,3].(Staudinger liked to use the overarching term “macromolecules ”but to note polymer therapeutics discussed here natural,pseudo-synthetic and synthetic polymers as seen as the platform not biotech drugs and delivery systems).His Nobel lecture also acknowledged that “.....macromolecular chemistry appears today to fit between low molecular organic chemistry and cytology.....”[2],wise words indeed given our continuing interdisciplinary attempts to treat diseased cells using polymer-based therapeutics often designed to navigate their endocytic pathways for lysosomotropic or endosomotropic (cytosolic)drug delivery [4,5].Just as the turn of the last century witnessed landmark changes in our understanding of macromolecular chemistry (Staudinger [2]),phagocytosis (Mechnikov [6]),and the poten-tial of synthetic low molecular weight chemotherapy and drug targeting (Ehrlich [7]),the beginnings of the 21st century have been equally inspir-ing.The last decade has witnessed some remarkable advances in the un-derstanding of the molecular basis of many diseases [8],the molecular basis of the endocytic machinery in health and disease [9],and not least,in nano-and material-science focused towards medical applications —the birth of “nanomedicine ”as a new discipline (reviewed in Ref.[1]and references therein).Thus the landscape for design,preclinical evaluation,and clinical use of polymer therapeutics is rapidly chang-ing and future successes will rely on our ability to embrace all theemerging opportunities and apply them to design and development of the next generation technologies.It would be inappropriate to simply revisit all of the topics/prima-ry papers that we have already comprehensively reviewed in recent reviews published in this journal and elsewhere (see Refs.[10–19]).Therefore here,and in the spirit of this “birthday ”issue,we have col-lated some of the key points to illustrate the historical journey of polymer therapeutics from lab to clinic,to brie fly discuss the current status,and most importantly try to highlight some of the emerging opportunities and challenges facing polymer therapeutics today,and in the foreseeable future.It is interesting to consider how poly-mer therapeutics might be used clinically as this century progresses and what percentage of the therapeutic market will they hold?To note,this short overview relies heavily on these recent key reviews as a reference point,so the reader is directed to their extensive bibliogra-phy for a more comprehensive listing of landmark primary papers.1.2.Evolution of polymer therapeuticsSince the 1940s synthetic polymers have been explored as therapeu-tics with a dramatic increase in publications year on year (Fig.1b).The number of papers in 2011interestingly rose to ~9.000,still some 10-fold(b)(a)Polymer-protein/ peptide conjugatesPolymeric drugsBlock copolymer micellePolymer-drug conjugatesPolymer DrugsImaging AgentLinkerProteinTargeting residueCoiled-coil peptideSelf assembling nano-sizedparticleFig.1.Polymer therapeutics.Panel (a)shows a schematic of the sub-classes of polymer therapeutic and panel (b)a comparative “pubmed ”literature search against the phrase “Polymer Therapeutic ”and the words “Dendrimer ”and “Nanomedicine ”(from Ref.[58]).61R.Duncan,M.J.Vicent /Advanced Drug Delivery Reviews 65(2013)60–70higher than is still seen for the descriptor“nanomedicine,”a wider disci-pline that is growing exponentially(a~300%increase in publications over the last3years).In the early years,polymers studied clinically were mainly plasma expanders(e.g.poly(vinylpyrolidone)(PVP)and dextran)and materials for use as wound dressings and antiseptics (PVP-iodine called Povidone).It is also noteworthy that polymers(e.g. high and low molecular weight dextran,dextrin and other oligosaccha-rides)have also been used as coatings,e.g.for colloidal iron nanoparticle “complexes”that are used to treat anaemia since the1930s,leading to a large clinical database on the safety of these products during parenteral administration.From the1960s synthetic polymer-based drugs,poly-mer–drug conjugates and the polymer,especially poly(ethyleneglycol) (PEG),-protein conjugates began to emerge.During the1970s interest in the lysosomotropic polymer–drug conjugates,block copolymer micelles,and PEG-protein conjugates began to grow exponentially due to the pioneering work of De Duve and colleagues[19],Ringsdorf and colleagues[20,21],and Davis and colleagues[22,23]respectively.Over the years PEGylation of proteins[24]and more recently aptamers[25] is producing an increasing number of important medical products.The concept of PEGylation is now widely accepted and it showed be emphasised that the success of PEG-proteins was critical in giving credi-bility to the wholefield of polymer therapeutics.At this time,and inspired by advances in cell biology[26,27]and poly-mer chemistry[20],we(RD)began the rational design of anticancer poly-mer–drug conjugates and a collaboration with Helmut Ringsdorf and then Jindrich Kopecek and colleagues at the Institute of Macromolecular Chemistry Prague which led to the N-(2-hydroxypropyl)methacrylamide (HPMA)-doxorubicin conjugates that became thefirst synthetic poly-mer–drug conjugates to enter Phase I/II clinical trials in1994.This story has been well documented[5,11,28–31],and also we have recent-ly critically reviewed the current status and future potential of HPMA copolymer-based therapeutics[14].In addition it should be noted that many of the preclinical models we established at Keele University in the1980s for evaluation of polymer-anticancer drug conjugates(e.g. for drug linker cleavage,determination of in vitro and in vivo toxicity, pharmacokinetics(PK),and efficacy,and also for determination of pre-clinical safety prior tofirst in man studies)have proved robust when transferred into other academic and industrial laboratories[29,31].In the1980s/90s important contributions of Kataoka and colleagues [32,33]and Kabanov and colleagues[34]led to the development of thefirst(advanced)block copolymer micelles to enter Phase I/II trials as novel anticancer agents.In the1990s(and after a spell in industry and exposure to the opinions of Regulatory colleagues)RD coined the term“polymer therapeutics”(as defined in Refs.[4,35])to better describe those polymer-based constructs viewed as“new chemical entities”rather than Drug Delivery Systems(DDS)per se that non covalently entrap a drug.The Centre for Polymer Therapeutics was established at the London School of Pharmacy,and today this term has become widely accepted with the initiation of Graduate Courses in Polymer Therapeutics,“Profes-sors of Polymer Therapeutics,”thefirst Polymer Therapeutics Laboratory has been established in Spain(MJV)with conferences dedicated specifi-cally to the topic.Often being complex,molecularly“engineered”con-structs of nano-size,polymer therapeutics have also been viewed as first generation“Nanomedicines”(discussed in Refs.[1,36]).At the outset,many dismissed all innovative“advanced drug delivery systems”as interesting but impractical scientific curiosities without any potential for commercial development.With this conservatism in the pharmaceutical sector,a business largely focussed(>90%)on the devel-opment of low molecular new chemical entities(NCEs as both innova-tive products and generics),and the lack of industrial experience in polymer chemistry and hybrid advanced drug delivery technologies in terms of manufacturing,formulation and the clinical protocols required for progress tofirst-in-man studies,thefirst product to market was in-evitably slow.Never the less over the years there have been an increas-ing number of clinical success stories across all thefirst generation “nanomedicine”technology areas,i.e.liposomes,nanoparticles and immunoconjugates(reviewed in Ref.[1]).All topics were discussed in Advanced Drug Delivery Reviews all those years ago.Today some still suggest that the lengthy timeframe needed for thefirst entry to market for each technology,and the relatively modest number of nanomedicine products that have so far successfully completed that journey constitute a failure.We would disagree as a touch of realism is needed.Given the creativity and persistence of all those early pioneers(academic and in-dustrial)who did manage to establish clinical proof of concept,despite the prevailing background doubts,it is perhaps more appropriate to ac-knowledge the progress as remarkable success story,and a valuable learning curve for nanomedicines yet to come.Thefirst advanced drug delivery systems are gaining an ever-increasing market share,and this in turn is stimulating much greater investment in second generation sys-tems across all the technology platforms-setting the scene for the future.In the area of polymer therapeutics,polymeric drugs[37,38], polymeric sequestrants[39],and PEG conjugates(both protein con-jugates[16]and the PEG-aptamer conjugate(Macugen)[40])have progressed to market(for examples see Table1).Moreover,there is a healthy clinical development pipeline in all the sub-classes shown in Fig.1a.Particular success stories include Copaxone as a treatment for multiple sclerosis(a complex random copolymer of three amino acids), the PEGylated interferons(Pegasys;Peg-Intron),and the PEGylated rhG-CSF(Neulasta)as a more convenient once-a-cycle adjunct to cancer chemotherapy.The orally administered polymeric sequestrants Renagel and Welchol are a particularly impressive example having progressing rapidly from concept to both clinically and economically important prod-ucts-from idea to GelTex Pharmaceuticals to Genzyme to Sanofiin a very short time frame!It is disappointing however that despite almost two decades of clin-ical development involving polymer–drug conjugates and(advanced) block copolymer micelles,both being largely explored clinically as novel anticancer agents,that these sub-classes have yet to realise a first marketed product.There is a significant pipeline in Phase II/III development so this important landmark cannot be far away.The slow progress in some ways parallels that seen for anticancer immu-noconjugates.Both share the challenges of tumour targeting and linker optimisation for drug release.In the1980s dendrimers emerged as in-teresting new class of polymers.Although they present appealing ad-vantages when compared to more traditional branched and linear polymers(theoretically better control of composition,3D architecture and a multi-functionality)they have proved very difficult to progress to the clinical stage of development.The only parenteral product to progress into clinical development has been the now discontinued blood pool MRI imaging agent Gadomer-17[41],but a topical gel in-cluding the lysine-based dendrimer virocide,Vivagel,is also in clinical development as a preventative agent for HIV infection.While a growing number of polymer therapeutics have shown abil-ity to satisfy the stringent requirements of“Regulatory Authority Approval,”many of the emerging technologies are more complex, often being multi-component systems containing targeting ligands, imaging agents and even multiple drugs for combination therapy. Self-assembling,supramolecular constructs are also becoming popular and control of reproducible“quality”is challenging especially if the components are mixed immediately before administration in the hospi-tal pharmacy.Careful process control during industrial scale manufac-ture and validated characterisation of the end products is essential to ensure batch-to-batch reproducibility.Heterogeneity can be acceptable but the specification set must adequately cover the critical product at-tributes governing safety,and efficacy.For polymer therapeutics impor-tant characteristics include molecular weight and polydispersity,size, architecture,and surface chemistry,including hydrophilicity,hydro-phobicity,and charge density.Variations in polymer tacticity[42],ste-reochemistry[43]and solution conformation[44],as well as the counterion of polyelectrolytes also play an important role in governing biological properties.The more complex the composition,the more challenging the validated characterisation-each component must be62R.Duncan,M.J.Vicent/Advanced Drug Delivery Reviews65(2013)60–70de finable in terms of identify and content (strength)in the presence of all the others.Moreover,as well as the low molecular weight impurities typical of any drug product,macromolecular impurities may also be present.Novel methodology is frequently required to carefully de fine composition and stability.Although polyglutamic acid (PGA)is being increasingly used as a drug carrier (reviewed in Ref.[18]),and a number of PGA-anticancer conju-gates are in clinical trials (e.g.[48]),most other parenteral conjugates in clinical development use either PEG or HPMA copolymers as the polymer platform.Although their molecular weight can be tailored to maximise the chance of renal elimination,these are non-biodegradable and thus have the potential to accumulate intracellularly,thus they present the risk of a “lysosomal storage disease ”syndrome (discussed in Ref.[19]).This is especially a concern when non-degradable polymers are used at high doses and/or repeatedly to treat indications where chronic parenter-al administration is required.Preclinical evidence of the induction of in-tracellular vacuolation by certain PEG-protein conjugates (reviewed in Ref.[49])has increased awareness of the potential problem,and the po-tential risk of any non-biodegradable polymer (used as a drug or compo-nent of a conjugate or supramolecular system)should be carefully considered for in respect of dose administered,frequency of dosing and clinical setting.This is leading to increasing interest in the use of bio-degradable polymers for new generation polymer therapeutics as discussed below.To address these challenges there is a growing utilisation of a “quality by design approach ”(QbD)to preclinical structure optimisation of polymer therapeutics and to manufacturing process con-trol and to give some background,the requirements are discussed in respect of low molecular weight chemical entities and biotech products in Refs.[45,46].The speci fic challenges for industrial development and Regulatory consideration relating to polymer therapeutics have been discussed in detail elsewhere [13].Some of the main points frequently discussed include:Quality•Always concerns regarding use of novel polymers or block co-polymers (not previously approved for use in man).There is aneed to consider critical attributes in respect of safety on a case by case basis.•De finition of,and minimisation of,heterogeneity within com-plex multicomponent products;even the simplest tripartite constructs comprise a polymer,linker,and drug.Others can ad-ditionally contain targeting residues,imaging agents and/or use of complex block copolymers or dendritic architectures.Control of synthetic methods is essential.•Control of manufacturing on the industrial scale to ensure an acceptable/reproducible speci fication must be achieved -scale-up manufacture and puri fication bring different chal-lenges compared to low molecular weight chemical enti-ties.•Development of an appropriate formulation (needs relate to route of administration),to ensure stability on storage and appropriate form at point of administration (e.g.absence of particulates during intravenous (i.v.)administration).•Development of validated analytical techniques to character-ise the polymer therapeutic (drug),the formulation of the polymer therapeutic (drug product),and its stability.•Understanding the distinction between the “polymer thera-peutic ”(drug)and the excipients (also potentially polymeric)used as addition during formulation development.Safety and ef ficacy•To ensure meaningful results,only well-characterised poly-mer therapeutics should be used for all biological studies.•De finition of and optimisation of the critical product attri-butes controlling safety and ef ficacy.•Understanding that the ADME (administration,distribution,metabolism and elimination)of polymer therapeutics will be very different from a low molecular weight chemical entity (reviewed in Ref.[47]).Table 1Examples of polymer therapeutics in the market and clinical development.a Sub class ExamplesCompositionStatusPolymeric drugsCopaxone Glu,Ala,Tyr copolymer Market VivagelLysine-based dendrimer Phase III Hyaluronic acid Hyalgal,SynviscMarket Polymeric sequestrants Renagel WelcholPhosphate binding polymer cholesterol bindingMarket MarketPolymer –protein conjugates Zinostatin Stimaler Styrene maleic anhydride-neocarzinostatin,(SMANCS)Market (Japan)SuliXen Polysialylated insulin Phase I/II PEGylated proteinsCimzia PEG-anti-TNF Fab Market Mircera PEG-EPOMarket Peg-intron PEG-Interferon alpha 2b Market Pegasys PEG-Interferon alpha 2a Market NeulastaPEG-hrGCSF Market Uricase-PEG 20PEG-uricaseMarket ADI-PEG 20PEG-arginine deaminase Phase II PEGylated-aptamerMacugen PEG-aptamer (apatanib)Market E10030PEG-anti-PDGF aptamerPhase II ARC1779PEG-anti-platelet-binding function of von Willebrand Factor Phase II Polymer –drug conjugateCT-2103;Xyotax;Opaxio Poly-glutamic acid (PGA)-paclitaxel Phase II/III Prolindac HPMA –copolymer –DACH platinatePhase II PEG-SN38Multiarm PEG-camptothecin derivative Phase II XMT-1001Polyacetal-camptothecin conjugate Phase I NKTR-118PEG-naloxonePhase III Block copolymer micellesSP1049C Doxorubicin block copolymer micelle Phase I/II NK 105Paclitaxel block copolymer micelle Phase II NK-6004Cisplatin block copolymer micellePhase II Self assembled polymer conjugate nanoparticlesIT-101Polymer conjugated-cyclodextrin nanoparticle-camptothecin Phase II CALAA 01Polymer-conjugated cyclodextrin-nanoparticle-siRNAPhase IaSee Ref.[1]for a comprehensive list of polymer therapeutics and other nanomedicine products in clinical use and clinical development and Ref.[10]for further details on on-going clinical programmes.63R.Duncan,M.J.Vicent /Advanced Drug Delivery Reviews 65(2013)60–70•Establishing and validating new in vitro and in vivo methodsto adequately characterise the critical product attributes inpreclinical development.•Design of preclinical safety studies on a product by product,route of administration and clinical setting basis to addressany polymer therapeutic-specific safety issues.•Development of appropriate in vitro and in vivo models todefine efficacy.Models should be validated for the relevantbiomarkers of polymer therapeutic performance(not justreliance on existing models typically used to assess low mo-lecular weight chemical entities exhibiting a totally differ-ent cellular and whole body pharmacokinetics(PK)).2.The futureGiven the current drug attrition rates(up to95%of all agents en-tering clinical trial fail-for all agents)it is always dangerous to try and predict“the future”in medicines development.It is easy to un-derestimate the degree of difficulty,but equally easy to underesti-mate the potential for rapid progress when scientific advances are strategically applied to a medical need,cf.Welchol and Renagel. Clearly the last decade has seen significant progress in the basic sci-ences underpinning polymer therapeutic design.In parallel,the Reg-ulatory Sciences underpinning commercialisation have also matured and more and more products are coming into clinical development and to the market in timely fashion.This bodes well for the future. Over the next decade it seems certain that an increasing number of polymeric drugs,polymer–protein and polymer–aptamer conjugates will enter the market and that thefirst polymer–drug and block co-polymer micelle products will make this transition.As for the other sub-classes,it is likely that afirst commercial success in these areas will dramatically increase confidence in the technology per se and more products will quickly follow.Over the last3decades the most challenging domain for all advanced drug delivery systems(including viral vectors)has been realisation of efficient intracellular delivery of genes,biologics and other macromolecular drugs.Design of non-viral vectors(including polymer therapeutics)for cytosolic delivery,has undergone something of a renaissance in recent years due to the growing interest in siRNA as a therapeutic.However,it is still far from clear whether successful exploitation of non-viral vectors designed for cytosolic delivery will be achieved in the near future. The failure so far of gene therapy(even using viral vectors),antisense and ribozyme therapeutics illustrate the ongoing challenges which relate to both the molecular mechanisms of the therapeutic itself as well as the complexities of delivery system design.We have recently reviewed all aspects of polymer therapeutics [15–19]including polymer synthesis,linker chemistry,methods for characterisation,improved understanding of solution properties, definition in structure-activity relationships in complex biological en-vironments,and novel concepts for polymer therapeutics design (summarised in Table2).Here we summarise some of the key issues and spotlight some of the most exciting prospects for these technolo-gies in the near and longer term future.2.1.Chemistry and analytical toolsRecent advances in chemistry include new routes to linear syn-thetic polymers including reversible addition-fragmentation chain transfer polymerisation(RAFT),atom transfer radical polymerisation (ATRP)and“one-pot”synthesis techniques to hyperbranched poly-mers.RAFT and ATRP offer the possibility to reduce heterogeneity, and give greater control over molecular weight average and polydis-persity.New opportunities for conjugation have also been developed including click chemistry and novel approaches to linker design have appeared,e.g.peptidyl linkers designed for cleavage by the tumour-associated protease legumain,self-immolative linkers,bioreductive linkers,and bioresponsive coiled-coil peptide linkers.Innovative syn-thetic methods are leading to a wide array of polymer architectures including block and graft copolymers,star polymers,dendrimers, dendrons and other complex dendritic architectures(reviewed in Refs.[17,18]).Moreover with the advent of“nano”it has become popular to use self-assembling macromonomers to create polymeric nanoparticles and polymerosomes.The latter are typically much larger(>30–50nm)than polymeric drugs or polymer conjugates that are similar in size to proteins(typically5–20nm).The self-assembling cyclodextrin-containing polymer conjugate-based nanoparticles(30–40nm)pioneered by Davis and colleagues haveTable2Emerging opportunities.Advances in polymer chemistry and new concepts.aPolymer,chemistry,novel concept Examples Status/productControlled polymerisation techniques Reversible addition-fragmentation chain transferpolymerisation(RAFT)and atom transfer radical polymerization(ATRP)Recombinant/genetic ligation of biodegradable polymers to proteins —an alternative toPEGylation XTEN polypeptide technologyAequus Glycopolymer technologyVRS-859for type II diabetes in Phase IVRS-317for growth hormone deficiencyin Phase IBiodegradable polymers Polyglutamic acid(PGA)Anticancer conjugates in Phase II/IIIHydroxyethyl starch(HES)Market as a plasma expanderDextrin Market as a peritoneal dialysis solutionPolyacetal(Fleximer)Anticancer conjugates in Phase I/IIPoly(sialic acid)Bioresponsive polymer–protein conjugates Polymer-masking-unmasking-protein therapy(PUMPT)Dextrin-PLA2in vitro proof of conceptDextrin-rhEGF in vivo proof of concept Combinations type1Polymer–drug conjugates+free drug Anticancer agents in Phase IIOpaxio+platinatesOpaxio+temozolamideOpaxio+radiotherapyType2Polymer–drug conjugate+polymer–drug conjugate In vitroType3Single polymer chain carrying more than1drug In vivoType4Polymer-directed enzyme prodrug therapy(PDEPT)Polymer–enzyme liposome therapy(PELT)In vivo In vitroSelf-assembling polymer therapeutic nanoparticles Cyclodextrin-containing polymer conjugate-basednanoparticles(30–40nm)Anticancer agents in Phase I/II CALAA01for siRNA deliveryIT-101for camptothecin deliverya See Refs.[12,17,18]for in depth details of these topics.64R.Duncan,M.J.Vicent/Advanced Drug Delivery Reviews65(2013)60–70。
硼酸酯键的英文
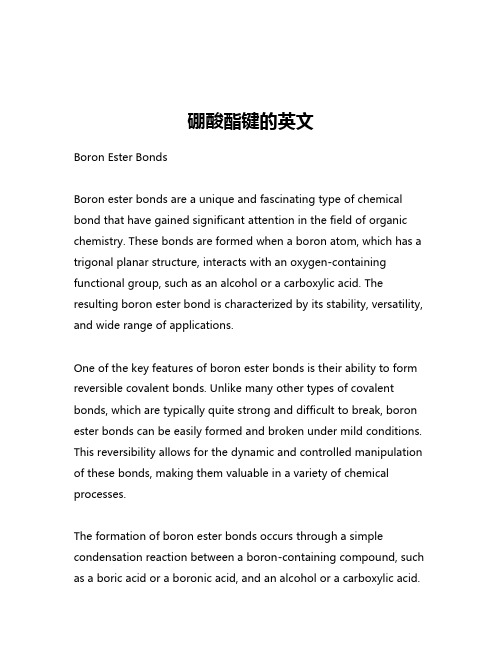
硼酸酯键的英文Boron Ester BondsBoron ester bonds are a unique and fascinating type of chemical bond that have gained significant attention in the field of organic chemistry. These bonds are formed when a boron atom, which has a trigonal planar structure, interacts with an oxygen-containing functional group, such as an alcohol or a carboxylic acid. The resulting boron ester bond is characterized by its stability, versatility, and wide range of applications.One of the key features of boron ester bonds is their ability to form reversible covalent bonds. Unlike many other types of covalent bonds, which are typically quite strong and difficult to break, boron ester bonds can be easily formed and broken under mild conditions. This reversibility allows for the dynamic and controlled manipulation of these bonds, making them valuable in a variety of chemical processes.The formation of boron ester bonds occurs through a simple condensation reaction between a boron-containing compound, such as a boric acid or a boronic acid, and an alcohol or a carboxylic acid.The reaction typically involves the displacement of water, resulting in the formation of the boron ester bond. This process can be catalyzed by the presence of an acid or a base, depending on the specific requirements of the reaction.One of the most remarkable applications of boron ester bonds is in the field of organic synthesis. These bonds can be used as versatile building blocks in the construction of complex organic molecules, allowing for the introduction of a wide range of functional groups and the formation of intricate molecular structures. Boron ester bonds have been employed in the synthesis of various natural products, pharmaceuticals, and other important organic compounds.Moreover, boron ester bonds have found utility in the development of novel materials and polymers. The reversible nature of these bonds allows for the creation of dynamic and responsive materials, such as self-healing polymers and stimuli-responsive gels. These materials can adapt to changes in their environment, exhibiting unique properties that are not achievable with traditional covalent bonds.In the field of biochemistry and biology, boron ester bonds have also garnered significant attention. Certain enzymes and biological molecules have been found to utilize boron ester bonds in their structure and function. For instance, some enzymes involved incarbohydrate metabolism have been shown to employ boron ester bonds to facilitate specific reactions. Additionally, boron-containing compounds have been investigated for their potential therapeutic applications, such as in the treatment of cancer and other diseases.The study of boron ester bonds has also led to the development of new analytical techniques and characterization methods. The unique properties of these bonds, such as their sensitivity to various environmental factors and their ability to form specific complexes, have enabled the design of specialized analytical tools for the detection and quantification of boron-containing compounds.In conclusion, boron ester bonds are a remarkable and versatile class of chemical bonds that have found widespread applications in various fields of chemistry, materials science, and biology. Their reversible nature, stability, and diverse functionalities have made them an important area of research and development, with the potential to contribute to numerous scientific and technological advancements in the years to come.。
JACS25位副主编的研究兴趣和实验室主页

JACS所有25位副主编列表:/page/jacsat/editors.htmlEric V. Anslyn: Supramolecular Analytical Chemistry, small molecule therapeutics/research/sm.htmlStephen J. Lippard: bioinorganic chemistry. The core activities include both structural and mechanistic studies of macromolecules as well as synthetic inorganic chemistry. The focus is on the synthesis, reactions, physical and structural properties of metal complexes as models for the active sites of metalloproteins and as anti-cancer drugs. Also included is extensive structural and mechanistic work on the natural systems themselves. A program in metalloneurochemistry is also in place./lippardlab/Weston Thatcher Borden: Computational Chemistry; Organic Chemistry; Organometallic Chemistry; Application of quantitative electronic structure calculations and qualitative molecular orbital theory to the understanding and prediction of the structures and reactivities of organic and organometallic compounds./people-node/weston-t-bordenThomas E. Mallouk: Chemistry of Nanoscale Inorganic Materials: Solar Photochemistry and Photoelectrochemistry; Nanowires; Functional Inorganic Layered Materials; In-Situ Remediation of Contaminants in Soil and Groundwater Using Nanoscale Reagents/mallouk/Benjamin F. Cravatt: Chemical Strategies for the Global Analysis of Enzyme Function; Technology Development: Activity-Based Protein Profiling (ABPP); Biological applications of ABPP - profiling enzyme activities in human cancer.; Advancing the ABPP technology; Technology Development: Protease Substrate Identification; Basic Discovery: The Enzymatic Regulation of Chemical Signaling /cravatt/research.htmlChad A. Mirkin: He is a chemist and a world renowned nanoscience expert, who is known for his development of nanoparticle-based biodetection schemes, the invention of Dip-Pen Nanolithography, and contributions to supramolecular chemistry. Our research focuses on developing strategic and surface nano-optical methods for controlling the architecture of molecules and materials on a 1-100 nm scale. Our researchers, with backgrounds ranging from medicine, biology, chemistry, physics and material science, are working together in solvingfundamental and applied problems of modern nanoscience. Research in the Mirkin laboratories is divided into the five areas listed below: Anisotropic Nanostructures; On-Wire Lithography (OWL); Dip-Pen Nanolithography; Organometallic Chemistry; Spherical Nucleic Acids/mirkin-group/research/Paul Cremer: works at the crossroads of biological interfaces, metamaterials, spectroscopy, and microfluidics. Biophysical and analytical studies are tied together through the employment of novel lab-on-a-chip platforms which enable high throughput/low sample volume analysis to be performed with unprecedented signal-to-noise. From neurodegenerative diseases to artificial hip implants, a huge variety of processes occur at biological interfaces. Our laboratory uses a wide variety of surface specific spectroscopy and microfluidic technologies to probe mechanisms of disease, build new biosensors against pathogens, and understand the molecular-level details of the water layer hugging a cell membrane. Research projects in the Cremer Group are divided into the five areas listed below. Click on your area(s) of interest to learn more. SFG of Water and Ions at Interfaces; Hofmeister Effects in Protein Solutions; Bioinorganic Chemistry and Biomaterial Properties of Lipid Bilayers; pH Modulation Sensing at Biomembranes; Metamaterialshttps:///cremer/Jeffrey S. Moore:Our research involves the synthesis and study of large organic molecules and the discovery of new polymeric materials. Most projects relate to one of three areas: new macromolecular architectures and their supramolecular organization; responsive polymers including self-healing materials; mechanochemical transduction. In general, our group uses the tools of synthetic and physical organic chemistry to address problems at the interface of chemistry and materials science. More in-depth information about our research can be found on our research page./Lyndon Emsley: NMRhttp://perso.ens-lyon.fr/lyndon.emsley/Lyndon_Emsley/Research.htmlKlaus Müllen: The group pursues a broad program of experimental research in macromolecular chemistry and material science. It has a wide range of research interests: from new polymer-forming reactions including methods of organometallic chemistry, multi-dimensional polymers with complex shape-persistent architectures, molecular materials with liquid crystalline properties for electronic and optoelectronic devices to the chemistry and physics of single molecules, nanocomposites or biosynthetic hybrids.http://www2.mpip-mainz.mpg.de/groups/muellenJean M. J. Fréchet:Our research is largely concerned with functional polymers, from fundamental studies to applications. The research is highly multidisciplinary at the interface of several fields including organic, polymer, biological, and materials chemistry. Chemical Engineering is also well represented with our research in energy-related materials and microfluidics./Eiichi Nakamura: Fascination to learn about the nature of the elements and molecules and to control their behavior goes back to ancient times. The research programs in our laboratories focus on the development of new and efficient synthetic reactions, new reactive molecules, and new chemical principles that will exert impact on the future of chemical, biological and material sciences. Under the specific projects listed below, we seek for the new paradigm of chemical synthesis and functional molecules. Discovery based on logical reasoning and imagination is the key term of our research and educational programs.http://www.chem.s.u-tokyo.ac.jp/users/common/NakamuraLabE.htmlGregory C. Fu: Transition Metal Catalysis; Nucleophilic Catalysis/research.htmlWilliam R. Roush:Our research centers around themes of total synthesis, reaction development and medicinal chemistry. Over 25 structurally complex, biologically active natural products have been synthesized in the Roush lab. These serve both as testing grounds for new methods and as inspiration for potential therapeutics.Our total synthesis projects are often attempted in parallel with reaction design. Synthetic applications of intramolecular Diels-Alder reactions and acyclic diastereoselective syntheses involving allylmetal compounds are of especial interest.Total synthesis and methods development interact synergistically toward the development of medicinally relevant compounds. Current targets of interest include chemotherapeutics built upon the exploitation of tumor cell metabolism, cystein protease inhibitors for treatment of parasitic diseases and diagnostic probes for the Scripps Molecular Screening Center./roush/Research.htmlMiguel García-Garibay:Our group is currently investigating the photochemical decarbonylation of crystalline ketones. Because the reactions take place in the solid state, they exhibit high selectivites that are not possible by the analogous solution reaction. From our experience, the solution photolysis yields many products, while there is often only one product in the solid. In order for the decarbonylation reaction to proceed in crystals, there are a few requirements forthe decarbonylation precursor: (1) The compound must be a crystalline solid. (2) There must be suitable radical stabilizing substituents present at both alpha centers./dept/Faculty/mgghome/Alanna Schepartz: The Schepartz laboratory develops chemical tools to study and manipulate protein–protein and protein–DNA interactions inside the cell. Our approach centers on the design of molecules that Nature chose not to synthesize--miniature proteins, ß-peptide foldamers, polyproline hairpins, and proto-fluorescent ligands--and the use of these molecules to answer biological questions that would otherwise be nearly impossible to address. Current topics include the use of miniature proteins to identify the functional role of discrete protein-protein interactions and rewire cellular circuits, the use of cell permeable molecules to image misfolded proteins or protein interactions in live cells, and the design of protein-like assemblies of ß-peptides that are entirely devoid of -amino acids./research/index.htmlMartin Gruebele:The Gruebele Group is engaged in experiments and computational modeling to study a broad range of fundamental problems in chemical and biological physics. A common theme in the experiments is the development of new instruments to interrogate and manipulate complex molecular systems. We coupled experiments with quantum or classical simulations as well as simple models. The results of these efforts are contributing to a deeper understanding of RNA and proteins folding in vitro and in vivo, of how vibrational energy flows around within molecules, of single molecule absorption spectroscopy, and of the dynamics of glasses./mgweb/Matthew S. Sigman: Our program is focused on the discovery of new practical catalytic reactions with broad substrate scope, excellent chemoselectivity, and high stereoselectivity to access novel medicinally relevant architectures. We believe the best strategy for developing new classes of catalysts and reactions applicable to organic synthesis is using mechanistic insight to guide the discovery process. This allows us to design new reaction motifs or catalysts in which unique bond constructions can be implemented furthering new approaches to molecule construction. An underlying theme to these methodologies is to convert relatively simple substrates into much more complex compounds allowing for access to known and novel pharmacaphores in a modular manner. This provides us the ability to readily synthesis analogs enabling us to understand the important structural features responsibility for a phenotypic response in a given biological assay. We are currently engaged in several collaborative projects to evaluate our compound collections for various cancer types at the Huntsman Cancer Institute atthe University of Utah and are engaged in follow-up investigations to identify improved compounds as well as understanding the mechanism of action. The group is engaged in the following diverse projects:/faculty/sigman/research.htmlSidney M. Hecht: Sidney M. Hecht, PhD, is the co-director for the Center for Bioenergetics in the Biodesign Institute at Arizona State University. He researches diseases caused by defects in the body's energy production processes. Energy production is similar mechanistically to other molecular processes that he has studied extensively. Hecht played a key role in the development of Hycamtin, a drug used to treat ovarian and lung cancer, as well as the study of the mechanism of the anti-tumor agent bleomycin./people/sidney-hechtDonald G. Truhlar: Theoretical and Computational ChemistryWe are carrying out research in several areas of dynamics and electronic structure, with a special emphasis on applying quantum mechanics to the treatment of large and complex systems. Dynamical calculations are being carried out for combustion (with a special emphasis on biofuel mechanisms) and atmospheric reactions in the gas phase and catalytic reactions in the condensed phase. Both thermal and photochemical reactions are under consideration. New orbital-dependent density functionals are being developed to provide an efficient route to the potential energy surfaces for these studies. New methods are also being developed for representing the potentials and for combined quantum mechanical and molecular mechanical methods, with a special emphasis in the latter case on improving the electrostatics. New techniques for modeling vibrational anharmonicity and for Feynman path integral calculations are also under development./truhlar/Joseph T. Hupp: Most research projects revolve around a theme of studying materials for alternative energy applications and other environmental issues. Due to the interdisciplinary nature of our research, we have many joint students with other researchers both at Northwestern and at other institutions./hupp/research.htmlHenry S. White: My colleagues and I are engaged in both experimental and theoretical aspects of electrochemistry, with diverse connections to analytical, biological, physical, and materials chemistry. Much of our current research is focused on electrochemistry in microscale and nanoscale domains./faculty/white/white.htmlTaeghwan Hyeon: The main theme of our research is synthesis, assembly, and applications of uniformly sized nanoparticles.http://nanomat.snu.ac.kr/index.php?mid=InterestsPeidong Yang: The Yang research group is interested in the synthesis of new classes of materials and nanostructures, with an emphasis on developing new synthetic approaches and understanding the fundamental issues of structural assembly and growth that will enable the rational control of material composition, micro/nano-structure, property and functionality. We are interested in the fundamental problems of electron, photon, and phonon confinement as well as spin manipulation within 1D nanostructures./index.php/research/interests/William D. Jones:Our research group has an interest in examining the reactions of homogeneous transition metal complexes with organic substrates with an emphasis on bond activation processes that are of potential interest to the chemical industry. We also are doing theoretical DFT modeling of this chemistry on our CCLab cluster/~wdjgrp/wdj_home.html#research下面是一些网友对部分副主编(部分已经不是了)的评价,没有罗列网友的ID了,一并表示感谢。
Stimuli-Responsive Polymer
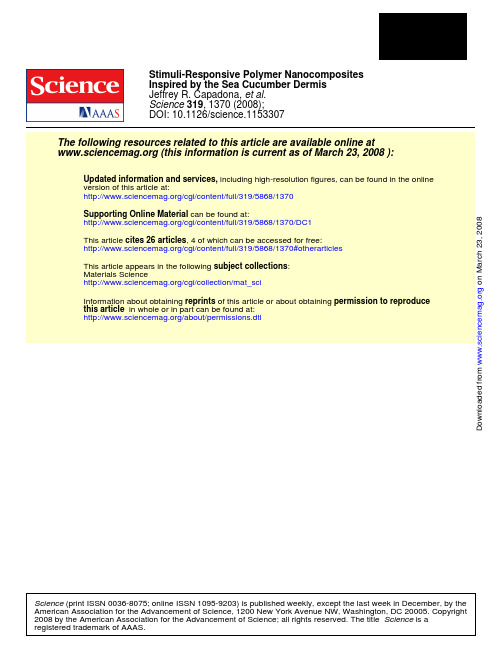
DOI: 10.1126/science.1153307, 1370 (2008);319Science et al.Jeffrey R. Capadona,Inspired by the Sea Cucumber Dermis Stimuli-Responsive Polymer Nanocomposites (this information is current as of March 23, 2008 ):The following resources related to this article are available online at/cgi/content/full/319/5868/1370version of this article at:including high-resolution figures, can be found in the online Updated information and services, /cgi/content/full/319/5868/1370/DC1 can be found at:Supporting Online Material /cgi/content/full/319/5868/1370#otherarticles , 4 of which can be accessed for free:cites 26 articles This article/cgi/collection/mat_sci Materials Science: subject collections This article appears in the following/about/permissions.dtl in whole or in part can be found at: this article permission to reproduce of this article or about obtaining reprints Information about obtaining registered trademark of AAAS.is a Science 2008 by the American Association for the Advancement of Science; all rights reserved. The title Copyright American Association for the Advancement of Science, 1200 New York Avenue NW, Washington, DC 20005. (print ISSN 0036-8075; online ISSN 1095-9203) is published weekly, except the last week in December, by the Science o n M a r c h 23, 2008w w w .s c i e n c e m a g .o r g D o w n l o a d e d f r o mprocesses in the fiber,in particular stimulated Raman scattering (20),limit the optical shock.Assuming that the steepness at the shock front is comparable to twice the frequency of the pulse carrier,8×1014Hz,the Hawking temperature (Eq.8)reaches 103K,which is many orders of magnitude higher than that in condensed-matter analogs of the event horizon (10–12,18).Our scheme thus solves two problems at once in a natural way:how to let an effective medium move at superluminal speed,and how to generate a steep velocity profile at the horizon.Here the various aspects of the physics of artificial black holes conspire together,in contrast to most other proposals (1–4,10–16).References and Notes1.Artificial Black Holes ,M.Novello,M.Visser,G.E.Volovik,Eds.(World Scientific,Singapore,2002).2.G.E.Volovik,The Universe in a Helium Droplet (Clarendon Press,Oxford,2003).3.W.G.Unruh,R.Schützhold,Quantum Analogues:From Phase Transitions to Black Holes and Cosmology (Springer,Berlin,2007).4.W.G.Unruh,Phys.Rev.Lett.46,1351(1981).5.T.Jacobson,Prog.Theor.Phys.136(suppl.),1(1999).6.G.Rousseaux,C.Mathis,P.Maïssa,T.G.Philbin,U.Leonhardt,/abs/0711.4767.7.S.M.Hawking,Nature 248,30(1974).8.N.D.Birrell,P.C.W.Davies,Quantum Fields in Curved Space (Cambridge Univ.Press,Cambridge,1984).9.R.Brout,S.Massar,R.Parentani,Ph.Spindel,Phys.Rep.260,329(1995).10.L.J.Garay,J.R.Anglin,J.I.Cirac,P.Zoller,Phys.Rev.Lett.85,4643(2000).11.S.Giovanazzi,C.Farrell,T.Kiss,U.Leonhardt,Phys.Rev.A 70,063602(2004).12.S.Giovanazzi,Phys.Rev.Lett.94,061302(2005).13.R.Schützhold,W.G.Unruh,Phys.Rev.D 66,044019(2002).14.G.E.Volovik,J.Exp.Theor.Phys.Lett.76,240(2002).15.U.Leonhardt,P.Piwnicki,Phys.Rev.Lett.84,822(2000).16.U.Leonhardt,Nature 415,406(2002).onni,Fast Light,Slow Light and Left HandedLight (Institute of Physics,Bristol,UK,2004).18.T.A.Jacobson,G.E.Volovik,Phys.Rev.D 58,064021(1998).19.R.Schützhold,W.G.Unruh,Phys.Rev.Lett.95,031301(2005).20.G.Agrawal,Nonlinear Fiber Optics (Academic Press,SanDiego,CA,2001).21.W.H.Reeves et al .,Nature 424,511(2003).22.P.Russell,Science 299,358(2003).23.G.t'Hooft.Nucl.Phys.B 256,727(1985).24.T.Jacobson,Phys.Rev.D 44,1731(1991).25.See the supporting material on Science Online.26.U.Leonhardt,Rep.Prog.Phys.66,1207(2003).27.Few-Cycle Laser Pulse Generation and Its Applications ,F.X.Kärtner,Ed.(Springer,Berlin,2004).28.T.Brabec,F.Krausz,Rev.Mod.Phys.72,545(2000).29.We are indebted to G.Agrawal,M.Dunn,T.Hänsch,ler,R.Parentani,and W.Sibbett for discussions and technical support.We thank A.Podlipensky and P.Russell for measuring the dispersion of our fiber.Our work was supported by the Leverhulme Trust,Engineering and Physical Sciences Research Council,Continuous Variable Quantum Information with Atoms and Light,the Ultrafast Photonics Facility at St Andrews,and Leonhardt Group Aue.Supporting Online Material/cgi/content/full/319/5868/1367/DC1SOM TextFigs.S1to S13Table S1References and Notes30November 2007;accepted 24January 200810.1126/science.1153625Stimuli-Responsive Polymer Nanocomposites Inspired by the Sea Cucumber DermisJeffrey R.Capadona,1,2,3Kadhiravan Shanmuganathan,1Dustin J.Tyler,2,3Stuart J.Rowan,1,2,3,4*Christoph Weder 1,2,4*Sea cucumbers,like other echinoderms,have the ability to rapidly and reversibly alter the stiffness of their inner dermis.It has been proposed that the modulus of this tissue is controlled by regulating the interactions among collagen fibrils,which reinforce a low-modulus matrix.We report on a family of polymer nanocomposites,which mimic this architecture and display similar chemoresponsive mechanic adaptability.Materials based on a rubbery host polymer and rigid cellulose nanofibers exhibit a reversible reduction by a factor of 40of the tensile modulus,for example,from 800to 20megapascals (MPa),upon exposure to a chemical regulator that mediates nanofiber ing a host polymer with a thermal transition in the regime of interest,we demonstrated even larger modulus changes (4200to 1.6MPa)upon exposure to emulated physiological conditions.Many echinoderms share the ability to rapidly and reversibly alter the stiffness of their connective tissue (1).In thecase of sea cucumbers (Fig.1,A and B),this morphing occurs within seconds and creates con-siderable survival advantages (1,2).A series of recent studies on the dermis of these invertebrateshas provided evidence that this defense mecha-nism is enabled by a nanocomposite structure in which rigid,high-aspect ratio collagen fibrils reinforce a viscoelastic matrix of fibrillin micro-fibrils (2–4).The stiffness of the tissue is regu-lated by controlling the stress transfer between adjacent collagen fibrils through transiently established interactions (5,6).These interactions are modulated by soluble macromolecules that are secreted locally by neurally controlled effec-tor cells.The dermis of the Cucumaria frondosa and other sea cucumber species represents a compelling model of a chemoresponsive material in which a modulus contrast by a factor of 10(~5to ~50MPa)is possible (7).Intrigued by this capability and with the goal of creating new dynamic materials for biomedical applications,we set out to investigate whether nanocompositescan be created that exhibit similar architecture and properties.The control of nanofiber inter-actions exploited here in solid polymer materials is similar to that observed in aqueous dispersions of poly(acrylic acid)-coated carbon nanotubes (8)or cellulose nanofibers (9),which have been shown to exhibit large viscosity changes upon variation of pH.The materials further comple-ment other polymeric systems with morphing mechanical behavior —for example,cross-linked polymers that change cross-link density upon a change in pH or ionic concentration (10,11).The first series of nanocomposites studied is based on a rubbery ethylene oxide –epichlorohydrin 1:1copolymer (EO-EPI)(Fig.1C)into which a rigid cellulose nanofiber network was incorpo-rated (Fig.1,C and D).The EO-EPI matrix dis-plays a low modulus and can accommodate the uptake of several chemical stimuli.Cellulose nano-fibers,isolated for this study from the mantles of sessile sea creatures known as tunicates (12),were used as the reinforcing component.These “whiskers ”exhibit high stiffness (tensile modu-lus ~143GPa)(13)and dimensions at the nano-meter scale (26nm ×2.2m m)(fig.S1).Similar nanofibers can be obtained from a range of re-newable biosources,including wood and cotton.Whiskers from tunicates were used here because their aspect ratio is higher than that of cellulose from other sources,which is advantageous for the formation of percolating architectures.Because of the high density of strongly interacting surface hydroxyl groups,cellulose whiskers have a strong tendency for aggregation (9,14).The whisker-whisker interactions can be moderated by the in-troduction of sulfate surface groups (Fig.1C),which promote dispersibility in select hydrogen-bond –forming solvents (14,15).This balance of attractive and repulsive interactions is the key for the fabrication of cellulose-whisker nanocomposites.1Department of Macromolecular Science and Engineering,Case Western Reserve University,Cleveland,OH 44106,USA.2Rehabilitation Research and Development,Louis Stokes Cleveland DVA Medical Center,10701East Boulevard,Cleveland,OH 44106,USA.3Department of Biomedical Engineering,Case Western Reserve University,Cleveland,OH 44106,USA.4Department of Chemistry,Case Western Reserve University,10900Euclid Avenue,Cleveland,OH 44106,USA.*To whom correspondence should be addressed.E-mail:christoph.weder@ (C.W.);stuart.rowan@ (S.J.R.)7MARCH 2008VOL 319SCIENCE1370REPORTSo n M a r c h 23, 2008w w w .s c i e n c e m a g .o r g D o w n l o a d e d f r o mGood dispersion is achieved during processing when whisker self-interactions are “switched off ”by competitive binding with a hydrogen-bond –forming solvent (Fig.1D)(14,15).Upon evap-oration of the solvent,the interactions among thewhiskers are “switched on,”and they assemble into a percolating network.This architecture and strong interactions among the whiskers maximize stress transfer and therewith the overall modulus of the nanocomposite (13,15,16).Similar to the seacucumber dermis,it should be possible to dynam-ically alter the modulus of the nanocomposites through the addition or removal of a chemical regulator that,in this case,would alter the extent of hydrogen bonding of the whiskers.3OAcnOOClxy nCellulose whisker EO-EPI PVAcInteraction “on”A B CFig.1.Natural model and bioinspired design ofchemomechanical nanocomposites.Pictures of a sea cucumber in relaxed (A )and stiffened (B )state demonstrating the firming of dermal tissue in the vicinity of the contacted area.(C )Chemical structure of cellulose whiskers isolated through sulfuric acid hydrolysis of tunicate cellulose pulp and the EO-EPI and PVAc matrix polymers used.(D )Schematic representation of the architecture and switching mechanism in the artificial nano-composites with dynamic mechanical properties.In the “on ”state,strong hydrogen bonds between rigid,percolating nanofibers maximize stress transfer and therewith the overall modulus of the nanocomposite.The interactions are switched “off ”by the introduction of a chemical regulator that allows for competitive hydrogen bonding.10101010E '(P a )Volume fraction filler ABC0.20% S o l v e n t U p t a k e (v /v )Volume fraction fillerVolume fraction filler0.2010101010E ' (P a )Fig.2.EO-EPI/whisker nanocomposites.(A )Tensile storage moduli E ′of EO-EPI/whisker nanocomposites as a function of volume fraction of cellulose whiskers.The nanocomposites were conditioned by either drying in vacuum,equilibrium swelling in deionized water,or swelling to saturation in de-ionized water followed by redrying in vacuum.Lines represent values predicted by the percolation and Halpin-Kardos model.The arrow indicates changes in modulus and volume fraction of whiskers resulting from aqueous swelling of one selected sample (19%v/v whiskers).(B )Solvent uptake as a function of whisker volume fraction under ambient conditions,immersion in deionized water,or isopropanol at room temperature.(C )Tensile storage moduli E ′of IPA-swollen EO-EPI/whisker nanocomposites as a function of volume fraction of cellulose whiskers.Lines represent values predicted by the percolation and Halpin-Kardos model.Data points represent averages (number of individual measurements,N ,=3to 6)±standard error measurements. SCIENCE VOL 3197MARCH 20081371REPORTSo n M a r c h 23, 2008w w w .s c i e n c e m a g .o r g D o w n l o a d e d f r o mEO-EPI/whisker nanocomposites were pro-duced by solution casting from dimethylform-amide (DMF),as previously reported (16),and the whisker content was varied between 0and 19%v/v.The thermomechanical properties of these materials were established by dynamic mechanical analyses (DMA)and tensile tests.DMA temperature sweeps (figs.S2and S3)display a glass transition temperature (T g )around –37°C (maximum of loss tangent,tan d ),which is independent of the whisker content and matches the T g of the neat EO-EPI matrix (fig.S2).The intensity of tan d decreases more than proportionally with the whisker concentra-tion (fig.S2),which is indicative of attractive polymer-whisker interactions.Figure 2A shows the tensile storage moduli (E ′)of dry EO-EPI/whisker nanocomposites extracted from the DMA traces for a temperature of 25°C,that is,in the rubbery regime far above T g .E ′increased with the whisker content from ~3.7MPa (neat polymer)to ~800MPa (19%v/v whiskers).The observed reinforcement suggests the formation of a percolating nanofiber network in which stress transfer is facilitated by hydrogen-bonding be-tween the whiskers.This hypothesis is supported by calculations obtained using a percolation model (16).Within the framework of the model,the tensile storage modulus of the nanocomposites (E ′)can be expressed as (17,18)E ′¼ð1−2y þy X r ÞE ′s E ′r þð1−X r Þy E ′r 2ð1−X r ÞE ′r þðX r −y ÞE ′swithy ¼X rX r −X c 1−X c0:4where E ′s and E ′r are the experimentally deter-mined tensile storage moduli of the neat EO-EPI (3.7MPa)and a neat tunicate whisker film (4.0GPa),respectively;y is the volume fraction of whiskers that participate in the load transfer;X r is the volume fraction of whiskers;and X c is the critical whisker percolation volume fraction calculated by 0.7/A .A is the aspect ratio of the whiskers and has a value of 84as determined by analysis of transmission electron microscopy (TEM)images (fig.S1).Figure 2A shows that the experimentally determined E ′values of dry EO-EPI/whisker nanocomposites agree with values obtained from Eq.1.By contrast,the data deviate strongly from the Halpin-Kardos model (fig.S4).This behavior is indicative for the formation of a percolating network of strongly interacting cellulose whiskers within the EO-EPI matrix (15,16).This architecture is confirmed by atomic force microscopy (AFM)(Fig.3A)and scanning electron microscopy (SEM)(Fig.3B)images,which both show that the cellulose whiskers form a percolating network within the EO-EPI matrix.Stress strain curves (fig.S5)reveal that the formation of a percolating networkof cellulose whiskers within the EO-EPI matrix not only affects E ′but also has a considerable influence on the maximum tensile strength (s ),which increased from 0.27±0.04(neat EO-EPI,stress at break)to 1.71±0.23MPa (14.3%v/v whiskers,stress at yield),whereas the elongation at break was reduced from 360±20to 6.7±0.8%(table S1).In view of the outstanding dispersibility of sulfate-modified cellulose whiskers in water (15),we elected to explore whether water could act as a chemical regulator for the whisker-whisker interactions in the EO-EPI/whisker nanocompos-ites.The atmospheric water uptake of the ma-terials is negligible under ambient conditions,that is,if not placed in an aqueous medium (Fig.2B).Dry EO-EPI/whisker nanocomposites were immersed in deionized water for 48hours to achieve equilibrium swelling (Fig.2B).Under these conditions,all compositions investigated exhibit modest aqueous swelling (~30%v/v),in-dicating that in the case of these compositions the water uptake is mainly governed by the matrix polymer with only minor variations due to whisk-er content.The tensile storage moduli for water-swollen EO-EPI/whisker nanocomposites were measured by DMA at 25°C in de-ionized water.A pronounced reduction of E ′compared with the dry nanocomposites can be observed (Fig.2A).The greatest mechanical contrast is seen in the case of the nanocomposite with the highest whisker content (nominally 19%v/v)(19),where E ′was reduced from ~800to 20MPa upon equilibrium swelling.At the same time,swelling with water leads to a significant decrease of the tensile strength (1.71±0.23to 0.37±0.11MPa for a 14.3%v/v whisker nanocomposite)(fig.S5and table S1)and an increase of the elongation at break (6.7±0.8to 17.8±0.39%).Control ex-periments with the neat EO/EPI (fig.S5and table S1)show minimal changes in tensile strength upon deionized water swelling.One argument that could be made against the interpretation that the observed changes inmodulus,elongation at break,and tensile strength are the result of switching off the nanofiber-nanofiber interactions is that simple swelling of the matrix alone could lead to a plasticizing ef-fect;however,careful analysis of our data shows that this is not the case.DMA traces (fig.S2)indicate that the EO-EPI/whisker nanocompos-ites do not undergo any phase transition that would lead to a drop in modulus,such as cross-linked polymer hydrogels and hygroscopic poly-mers,which can display a decrease of the glass transition temperature upon water uptake (20).Although E ′s of the neat EO-EPI is reduced from 3.7to 0.8MPa upon equilibrium swelling with water (Fig.2A),analysis in the context of the percolation model (Eqs.1and 2and fig.S7)shows that a reduction of E ′s alone cannot ac-count for a significant reduction of E ′.Figure 2A also reveals that even after correcting X r for water uptake,the percolation model no longer ade-quately describes E ′of the water-swollen nano-composites.By contrast,the moduli now are in much closer agreement with the Halpin-Kardos model (21),which has successfully been used to describe the modulus of nanocomposites in which the filler is homogeneously dispersed in a polymer matrix and does not display pro-nounced filler-filler interactions (22).The model assumes that the materials are equivalent to many layers of unidirectional plies oriented in alter-nating directions (–45°,0°,45°,and 90°),and the properties of the unidirectional reference ply are predicted by the Halpin-Tsaïequations where the tensile storage modulus in the longitudinal (E ′L )and transverse (E ′T )directions are given by (22,23)E ′L =E ′s [1+2(A )h L f r ]/(1−h L f r )and E ′T =E ′s [1+2h T f r ]/(1−h T f r )Thus,all data indicate that the stiffness reduction achieved in the EO-EPI/whisker nano-composites is related to the decoupling of the stress-transferring rigid nanofiber networkuponFig.3.Morphology of EO-EPI/whisker nanocomposites.(A )Representative AFM phase image of an ultramicrotomed nanocomposite containing 9.5%v/v whiskers in EO-EPI.The inset shows an AFM phase image of a neat EO-EPI reference (horizontal scale bar =500nm,vertical scale bar =phase shift 120to 0°).The samples were briefly (10s)immersed in tetrahydrofuran and rinsed with IPA to partially dissolve the polymer at the surface of the sample and to expose the inner structure of the films.(B )Representative SEM image of an untreated nanocomposite containing 9.5%v/v whiskers in EO-EPI.(1)(2)(3)(4)7MARCH 2008VOL 319SCIENCE1372REPORTSo n M a r c h 23, 2008w w w .s c i e n c e m a g .o r g D o w n l o a d e d f r o mintroduction of water as a competitive hydrogen-bonding agent.Consistent with the proposed mechanism,the switching is fully reversible:The materials adapted their original stiffness upon drying (Fig.2A).To investigate the specificity of the switching mechanism,we investigated the effect of isopro-panol (IPA)as the swelling agent.IPA was selected because it swells neat EO-EPI to a similar degree as water (Fig.2B)but is incapable of dispersing cellulose whiskers (16).The nano-composites swelled upon immersion in IPA (Fig.2B)to a level similar to that of the composites in water;however E ′barely changed in comparison to the dry state (Fig.2C),and the data fit the percolation model.This result confirms that the chemomechanical response is largely a result of disruption of the whisker-whisker interactions and not just plasticization of the material.By contrast,EO-EPI is plasticized considerably upon IPA swelling (E ′drops from 3.6to 0.93MPa).This contrast highlights the most important advantage of the nanocomposite approach over simple plasticization of a neat polymer.Although plasticization through solvent uptake,which is inherent to the latter,is a nonspecific process,the responsive nanocomposites can be designed to display a response that is specific to the nature of the stimulus.In addition,the nanocomposite ap-proach provides the ability to increase the initial stiffness and strength of the material and allows for the use of host polymers that have no thermal transition in the temperature regime of interest,such as the EO-EPI matrix used here.We are interested in exploiting dynamic mechanical materials in biomedical applications,specifically as adaptive substrates for intracortical microelectrodes.These implants have the ability to record brain unit activity (24).Brain-machine interfaces that rely on these electrodes providesolutions to medical conditions such as Parkin-son ’s disease,stroke,and spinal chord injuries (25).One problem with current microelectrodes is that the signal quality usually degrades within a few months,making chronic applications chal-lenging (26).One hypothesis for the cause of possible failure,especially in recording applica-tions,is that the micromotion of rigid electrodes within the soft cortical tissue chronically inflicts trauma on the surrounding neurons (27).We hypothesize that a mechanically adaptive elec-trode could alleviate this problem,and we are thus interested in designing devices that are initially rigid to allow for penetration of the pia mater during implantation (28)but that soften slowly and without excessive expansion upon implantation in response to the chemical envi-ronment within the brain (for an emulation,see Movie S1).For this application,an initial E ′of >4GPa is desirable to allow for the insertion of an electrode with typical dimensions into the cortex (29).Because EO-EPI/whisker nanocomposites display a substantially lower E ′,we sought to combine the switching mechanism with a chem-ically influenced thermal transition.We discov-ered that nanocomposites based on poly(vinyl acetate)(PV Ac)(Fig.1C)and cellulose whiskers display such a “dual ”responsive behavior.Our data show that,upon exposure to physiological conditions,the materials undergo a phase tran-sition;in addition,the reinforcing whisker net-work is disassembled.DMA experiments (Fig.4A and fig.S8)reveal that the neat PV Ac dis-plays a T g around 42°C,that,just above phys-iological temperature.E ′s of the neat polymer is considerably reduced upon heating from room temperature (1.8GPa at 23°C)to above T g (0.39MPa at 56°C)(this corresponds to T g +16°C and marks the temperature at which E ′s is starting to level off).As evidenced by DMA data,the introduction of cellulose whiskers into PV Ac has only a minimal influence on T g in the dry state (Fig.4A and fig.S8).The thermal transition is sharpened,and the temperature at which E ′begins to drop is increased from ~25to >40°C.For certain biomedical applications,this effect is very desirable,because it prevents the thermally induced softening of the material just upon ex-posure to body temperature.As a consequence of the already rather high stiffness of the glassy PV Ac matrix,only a modest reinforcement is observed for the nanocomposites below T g (E ′=5.1GPa with 16.5%v/v whiskers)(fig.S8).However,a dramatic effect is observed above T g ,where E ′is increased from 1.0MPa for the neat polymer matrix up to 814MPa with 16.5%v/v whiskers (at 56°C).The experimental data above T g match well with the percolation model (fig.S8),which indicates that also in this series a percolating network of strongly interacting whiskers is formed (15,16,18).The nanocom-posites demonstrate significant swelling in both deionized water and artificial cerebrospinal fluid (ACSF).The solvent uptake increases with in-creasing whisker content and temperature (fig.S9),lowers the T g to below physiological tem-perature (19to 23°C)(fig.S10),and reduces E ′dramatically.For example,the E ′of a 16.5%v/v whisker nanocomposite above T g is reduced from 814MPa (dry)to 10.8MPa (water swollen;data are for 56and 37°C,respectively,that is,16°C above the respective T g ).As for the water-swollen EO-EPI/whisker nanocomposites,the moduli of the ACSF swollen PV Ac/whisker nanocompos-ites are better described by the Halpin-Kardos than the percolation model (fig.S8),again in-dicative of decoupling of the stress-transferring nanofiber network upon introduction of water.Exposure to brain tissue,simulated here by immersing the samples into ACSF and heating to25303540455055106107108109101016.5 % v/v whiskers 12.2 % v/v whiskers 8.1 % v/v whiskers 4.0 % v/v whiskers 0.8 % v/v whiskers 0.0 % v/v whiskersE ' (P a )Temperature (°C)E ' (M P a )Time(min)Temperature ramp ABFig.4.PVAc/whisker nanocomposites.(A )Tensile storage moduli E ′of dry films of PVAc/whisker nanocomposites as a function of temperature.The nanocomposites contain between 0and 16.5%v/v whiskers.(B )Time-dependent modulus decrease of neat PVAc and a 12.2%v/v PVAc/whisker nanocomposite upon immersion into ACSF and increasing the temperature from 23°C to 37°C.Lines represent time required for temperature to increase from 23°C to 37°C and isothermal control at 37°SCIENCE VOL 3197MARCH 20081373REPORTSo n M a r c h 23, 2008w w w .s c i e n c e m a g .o r g D o w n l o a d e d f r o ma physiological temperature of 37°C at ~2°C/min (30)(Movie S1),leads to a pronounced reduction of E ′.Whereas the neat PV Ac (dry E ′s =1.8GPa at 25°C)instantly softens under these conditions (Fig.4B),the E ′of the whisker-reinforced nano-composites (see Fig.4B for a 12.2%v/v nano-composite)is reduced slowly over a period of 15min.The whisker-reinforced nanocomposite dis-plays a much higher dry E ′(4.2GPa at 25°C)than the neat PV Ac,but both materials reach nearly identical moduli upon immersion in ACSF at 37°C (1.6MPa).Our data support a simple and versatile strat-egy for the creation of polymer nanocomposites,whose architecture and mechanical adaptability closely mimic the proposed architecture and ob-served response of the sea cucumber dermis.The mechanical properties of these chemoresponsive materials can selectively and reversibly be con-trolled through the formation and decoupling of a three-dimensional network of well-individualized nanofibers in response to specific chemical trig-gers.It will be interesting to explore whether the framework can be adapted to nonchemical trig-gers,for example,optical or electrical stimuli.References and Notes1.T.Heinzeller,J.Nebelsick,Eds.,Echinoderms (Taylor &Francis,London,2004).2.T.Motokawa,Comp.Biochem.Physiol.B 109,613(1994).3.F.A.Thurmond,J.A.Trotter,J.Exp.Biol.199,1817(1996).4.I.C.Wilkie,J.Exp.Biol.205,159(2002).5.J.A.Trotter,T.J.Koob,Matrix Biol.18,569(1999).6.G.K.Szulgit,R.E.Shadwick,J.Exp.Biol.203,1539(2000).7.J.A.Trotter et al .,Biochem.Soc.Trans.28,357(2000).8.J.C.Grunlan,L.Liu,Y.S.Kim,Nano Lett.6,911(2006).9.M.M.de Souza Lima,R.Borsali,Macromol.Rapid Commun.25,771(2004).10.J.A.Jaber,J.B.Schlenoff,J.Am.Chem.Soc.128,2940(2006).11.D.M.Loveless,S.L.Jeon,S.L.Craig,J.Mater.Chem.17,56(2007).12.P.S.Belton,S.F.Tanner,N.Cartier,H.Chanzy,Macromolecules 22,1615(1989).13.A.Sturcova,J.R.Davies,S.J.Eichhorn,Biomacromolecules 6,1055(2005).14.O.van den Berg,J.R.Capadona,C.Weder,Biomacromolecules 8,1353(2007).15.M.A.S.Azizi Samir,F.Alloin,A.Dufresne,Biomacromolecules 6,612(2005).16.J.R.Capadona et al .,Nat.Nanotech.2,765(2007).17.M.Takayanagi,S.Uemura,S.Minami,J.Polym.Sci.C 5,113(1964).18.N.Ouali,J.Y.Cavaillé,J.Pérez,J.Plast.Rubber Comp.Process.Appl.16,55(1991).19.Swelling increased the volume of the nanocompositesand reduced the volume fraction of whiskers,X r .For example,when a nanocomposite with X r =19%v/v was swollen with water,X r decreased to 14%.Therepresentation of data in Fig.2A considers this effect to allow for analysis by the Halpin-Kardos model.A direct comparison of dry versus wet composites for the same fiber loading is shown in fig.S6.20.J.Kunzelman,B.R.Crenshaw,C.Weder,J.Mater.Chem.17,2989(2007).21.J.C.Halpin,J.L.Kardos,J.Appl.Phys.43,2235(1972).22.P.Hajji,J.Y.Cavaillé,V.Favier,C.Gauthier,G.Vigier,pos.17,612(1996).23.h L =[(E lr /E ′s )–1]/[(E lr /E ′s )+2A ],and h T =[(E tr /E ′s )–1]/[(E tr /E ′s )+2].A is the aspect ratio of the whiskers,f is equal to the volume fraction of the phase,and the subscripts s and r represent the soft phase and the rigid phase,respectively.E lr is the longitudinal Young ’s Modulus (130GPa),and E tr is the transverse Young ’s Modulus (5GPa)of an individual cellulosewhisker (22).To determine the tensile storage modulus of the isotropic nanocomposite (E ′),E ′L and E ′T must beinserted into one equation using the Halpin-Kardos model:E ′=4U 5(U 1–U 5)/U 1where U 1=1/8(3Q 11+3Q 22+4Q 66);U 5=1/8(Q 11+Q 22–2Q 12+4Q 66);Q 11=E ′L /(1–n 12n 21);Q 22=E ′T (1–n 12n 21);Q 12=n 12Q 22=n 21Q 11;Q 66=G 12;n 12=f r n r +f s n s =0.3;G 12=G s (1+hf r )/(1–hf r );h =(G r /G s –1)/(G r /G s +1),n is the Poisson ’s ratio,G is the shear modulus,and G r =1.77GPa.24.D.M.Taylor,S.I.H.Tillery,A.B.Schwartz,Science 296,1829(2002).25.A.B.Schwartz,Annu.Rev.Neurosci.27,487(2004).26.R.Biran,D.C.Martin,P.A.Tresco,J.Biomed.Mater.Res.82A ,169(2007).27.W.L.C.Rutten,Annu.Rev.Biomed.Eng.4,407(2002).28.D.H.Szarowski et al .,Brain Res.983,23(2003).29.K.Najafi,J.F.Hetke,IEEE Trans.Biomed.Eng.37,474(1990).30.Materials and methods are available as supporting material on Science Online.31.We thank F.Carpenter for the photography of thesea cucumber and L.McCorkle,J.Johnson,and M.Hitomi for assistance with the SEM,AFM,and TEM experiments,respectively.Financial support from DuPont (Young Professor Award to C.W.),the L.Stokes Cleveland VAMC Advanced Platform Technology Center,an Ohio Innovation Incentive Fellowship (to K.S.),theDepartment of Veterans Affairs Associate Investigator Career Development Program (to J.C.),and the National Institutes of Health are gratefully acknowledged.The authors declare that they have no competing financial interest.Supporting Online Material/cgi/content/full/319/5868/1370/DC1Materials and Methods Figs.S1to 10Table S1References Movie S16November 2007;accepted 1February 200810.1126/science.1153307Heterogeneous Nucleation Experiments Bridging the Scale from Molecular Ion Clusters to NanoparticlesPaul M.Winkler,1Gerhard Steiner,1Aron Vrtala,1Hanna Vehkamäki,2Madis Noppel,3Kari E.J.Lehtinen,4Georg P.Reischl,1Paul E.Wagner,1Markku Kulmala 2*Generation,investigation,and manipulation of nanostructured materials are of fundamental and practical importance for several disciplines,including materials science and medicine.Recently,atmospheric new particle formation in the nanometer-size range has been found to be a global phenomenon.Still,its detailed mechanisms are mostly unknown,largely depending on the incapability to generate and measure nanoparticles in a controlled way.In our experiments,an organic vapor (n -propanol)condenses on molecular ions,as well as on charged and uncharged inorganic nanoparticles,via initial activation by heterogeneous nucleation.We found a smooth transition in activation behavior as a function of size and activation to occur well before the onset of homogeneous nucleation.Furthermore,nucleation enhancement for charged particles and a substantial negative sign preference were quantitatively detected.Condensational growth,evaporation,and heterogeneous chemistry are important phenomena in materials science,fluid dynamics,aerosol physics and technology,and atmospheric chemistry,including cloud micro-physics and cloud chemistry.A prerequisite for the start of condensation is homogeneous nu-cleation of new particles or the activation of preexisting particles by heterogeneous nuclea-tion.The latter can occur either on ions,soluble particles,or insoluble particles,and is energet-ically easier than homogeneous nucleation (1).Both particle formation processes are of fun-damental as well as practical importance andhave been the subject of investigations for more than a century (2).Important examples repre-senting the different processes are given by the use of the Wilson cloud chamber (3)in high-energy physics for the case of ions,the for-mation of cloud droplets in the troposphere for the case of soluble particles,and the occurrence of ice nucleation for the case of insoluble seed particles (4).Atmospheric observations suggest that the initial formation and growth are two uncoupled processes (5–7),and therefore the activation mechanism of small clusters is of vital importance.Understanding the formation and initial growth processes in detail is also crucial to control the production of nanomate-rials (8).In this paper,we present experimental results for the activation of molecular cluster ions,charged and neutral clusters,and nanometer-size particles having almost monodisperse size distributions,1Fakultät für Physik,Universität Wien,Boltzmanngasse 5,A-1090Wien,Austria.2University of Helsinki,Department of Physical Sciences,Post Office Box 64,00014University of Helsinki,Finland.3Institute of Physics,University of Tartu,18Ülikooli Street,50090Tartu,Estonia.4Department of Physics,University of Kuopio and Finnish Meteorological Institute,Post Office Box 1627,70211Kuopio,Finland.*To whom correspondence should be addressed.E-mail:markku.kulmala@helsinki.fi7MARCH 2008VOL 319SCIENCE1374REPORTSo n M a r c h 23, 2008w w w .s c i e n c e m a g .o r g D o w n l o a d e d f r o m。
Materials science and engineering, an introduction Chapter 17 Corrosion and Degradations of material
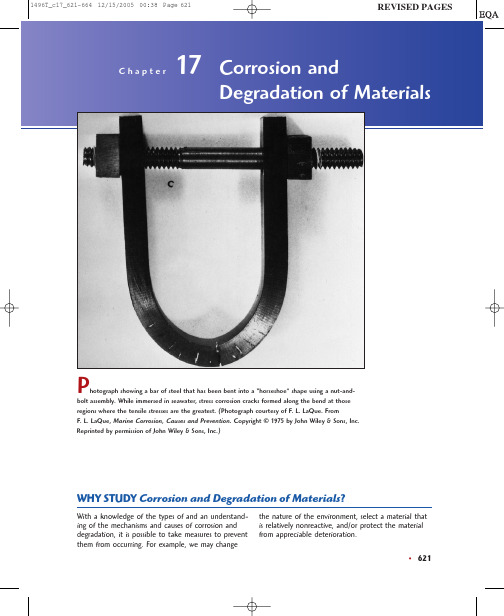
REVISED PAGES
Learning Objectives
After careful study of this chapter you should be able to do the following:
1. Distinguish between oxidation and reduction electrochemical reactions.
2. Describe the following: galvanic couple, standard half-cell, and standard hydrogen electrode.
3. Compute the cell potential and write the spontaneous electrochemical reaction direction for two pure metals that are electrically connected and also submerged in solutions of their respective ions.
7. List five measures that are commonly used to prevent corrosion.
8. Explain why ceramic materials are, in general, very resistant to corrosion.
9. For polymeric materials discuss (a) two degradation processes that occur when they are exposed to liquid solvents, and (b) the causes and consequences of molecular chain bond rupture.
博士申请用研究计划英文模板

pH-Responsive Biodegradable Polymers for Intracellular Drug DeliveryA. Proposed area of researchThe aim of this proposed PhD project is to develop and evaluate pH responsive, endosomolytic polymers for efficient intracellular delivery of biological drug payloads.There is a need to better understand the mechanisms of entry into the cell cytoplasm and nucleus in order to design optimal delivery systems for biological molecules. On the one hand, this would open up significant opportunities to deliver potent drug payloads against intracellular targets to positively impact human health. In addition the project aims to develop a more general understanding of the rules governing the uptake of biological molecules into cells.This project proposes to investigate the use of synthetic, biodegradable polymers for intracellular delivery of drug payloads (including siRNA, therapeutic peptide and antibody) against a well-validated intracellular drug target, such as Bcl-2. The novel pH- responsive polymers have been designed by Dr Rongjun Chen’sLab to mimic the activity of viruses, both in their cell entry and endosomal escape mechanisms. Using cancer cell lines (Jurkat or HL-60 cells) as a model system, the polymers would be tested with a variety of different biological payloads in a quantitative comparison of their ability to enter the cell and trigger apoptosis and subsequently cell death. With an efficient model system established, there would then be scope to optimize the systemin terms of the kinetics and mechanisms of cell entry, cytoplasmic and nuclear localization, and the biodegradation of the polymers. There would also be scope to explore the efficiency in other cell systems and with further intracellular targets. This multidisciplinary project is at the interface of Chemistry, Biology and Medicine, and will provide the student with a real opportunity to be involved in the development and evaluation of new nanomedicines.B. BackgroundAdvances in genomics and proteomics have enabled the development of macrodrugs, such as nucleic acids and proteins, with potential for the treatment of a wide variety of diseases. Amongst other problems, their clinical applications may be greatly impairedby low cellular uptake and lysosomal degradation before they can reach their target organelles or cell nuclei. In order to achieve efficient intracellular delivery of such biological molecules, delivery systems are required to enable high cell entry via endocytosis and efficient release into the cytoplasm by endosomal membrane disruption under mildly acidic conditions.Recombinant viruses and fusogenic viral peptides have been used to mediate gene transfection, but their clinical use is potentially limited by safety issues and difficultiesin large-scale production. A variety of synthetic polymers have therefore been developed as non-viral vectors. Cationic polyethyleneimine, poly(2- (dimethylamino)ethyl methacrylate) and polyamidoamine dendrimers mediate gene delivery through the ‘proton sponge ’effect, but suffer from c y y a t o n t d o x r e i c l i a t ivelylow transduction efficiencies. The intensively studied vinyl-based anionic polymers, poly(a-alkylacrylic acid)s, display pH-responsive membrane disruptive behavior, butthey are not biodegradable, thus low molecular weights have to be strictly required toallow renal excretion and their clinical applications are seriously limited.Dr Rongjun Chen’sLab has recently developed a class of novel, biodegradable, pH- responsive polymers to mimic factors that enable efficient viral transfection, but theyare safe, easy to manufacture and have more controllable structures. The parent polymer is a polyamide, poly(L-lysine isophthalamide), which was based on polycondensation of diacyl chlorides and natural metabolite tri-functional amino acids containing both - αa nd ω-amine groups. Hydrophobic amino acids and/or poly(ethylene glycol) were grafted onto its pendant carboxylic acid groups to manipulate its amphiphilicity and structure. The metabolite-derived biomimetic polymers can undergopH-mediated coil-globule changes in conformation. This property enables these polymers to be significantly membrane-disruptive within pH range typical of endosomal compartments, but necessarily non-toxic at physiological pH. Based on previous successful intracellular delivery of the model-drugs such as calcein, dextran (with molecular weight ranging from 3kDa to 70kDa), and therapeutic protein apoptin and siRNA, it is thought that these polymers may be able to deliver a wide variety of different biological molecules (nucleic acids and proteins) into cells for the treatment of various diseases including cancers.C. Applicant ’s work preparation in ChinaThe applicant is an expected bachelor majoring in Polymer Science and Engineering from Beijing University of Chemical Technology (BUCT). After four years of undergraduate studies (2007-2011), I have obtained a strong research background in organic chemistry, polymer physics and chemistry, physical chemistry, etc. Working inthe State Key Laboratory of Polymer Physics and Chemistry in the Institute of Chemistryof Chinese Academy of Science f or more than half of a year has set up my mind in researching polymer drug carriers. Our group cast our eyes towards synthesizing graft copolymers with amino acids as the main monomers, to create a novel carrier which isboth pH and temperature sensitive.We had synthesized a polymer brush from Z-lysine and 2-Bromoisobutyryl bromidethrough ring-opening polymerization. Then we grafted specific temperature sensitive residues onto the polymer brush via atomic transfer radical polymerization, followed by characterization and theoretical analysis of the polymers.In addition, I was a Research Assistant in the state key laboratory of Beijing University of Chemical Technology, working on the characterization of copolymers by NMR. I was alsoa Research Assistant in the Environmental Materials Laboratory of China Building Materials Academy, working on synthesis of FEVE coating. These research experiences have enriched my knowledge and experimental skills for polymer synthesis and enabledme to operate many facilities deftly, such as NMR, GPC,FTIR,vacuum glove box and rotary evaporator.In the summer of 2010, I was selected to attend the program -Cambridg“e S B u U m C m T e r School ”in the Ui v nersi t y of Cambridge. During the three weeks in the UK, I visited the Department of Chemical Engineering and Biotechnology and did experiments relevantto my research its labs. In Cambridge, I also did a case study about the biopharmaceutical market. This deepened my understanding of commercial prospectsof drug delivery technologies, such as the demands of different patients for drug delivery systems and competitiveness of different health testing equipments. Besidesthe University of Cambridge, I also visited the University of Oxford, Imperial College London, University of Birmingham, and University of Loughborough. I also establishedthe contact with Dr Rongjun Chen who is the Group Leader of Biomaterials and Drug Delivery Group at the University of Leeds when I was in the UK, and have been communicating with him via emails since then, discussing about polymer synthesis and characterization and drug delivery research.I believe the above mentioned academic backgrounds and various relevant experiences have prepared myself well for the PhD study on polymer drug delivery research for the treatment of various diseases including cancers in Dr Rongjun Chen’sLab at the University of Leeds.D. Aim of overseas studyThe aim of my PhD study is to apply polymer nanotechnology to drug delivery in orderto improve the safety and pharmaceutical efficacy of drugs that need precise intracellular delivery. I will design and synthesize biodegradable amino acid-based polymer vectors, which are efficient, safe, cost-effective and amenable to large-scale manufacturing. I will then evaluate the polymer-based targeted intracellular delivery of biological drug payloads (including siRNA, therapeutic peptide and antibody) against awell-validated intracellular drug target, such as Bcl-2, for cancer treatment. The intention is to combine the highly novel chemistry expertise surrounding the deliverypolymer with the biological expertise around the discovery and development of a variety of drug payloads. The novel polymer delivery technology to be developed will open the door to a wide variety of cytoplasmic and nuclear targets, previously thoughtto be inaccessible to biological therapy for various disease including cancers.In addition, I will investigate the fundamental mechanisms of the interaction between polymers/polymer-drug entities and different membrane models (artificial lipid membranes, erythrocytes and more complex nucleated mammalian cells) and obtain a better understanding of the rules controlling the uptake of macromolecules into cells.E. Research methodsThe project would break down into discrete stages as described below:(1) Polymer synthesisAmino acid derivatives (e.g. lysine derivative) will be used to carry out the N- Carboxyanhydride ring opening polymerization in order to obtain a polymer brush, which could be the backbone of target polymer. Then environmentally (e.g. pH and temperature) responsive groups will be grafted to the main chain by ATRP or Michael Addition, etc. Fluorescent polymers would be prepared by coupling organic fluorophores (e.g. fluorescein isothiocyanate and Cy5) onto the polymers. Cleavable linker chemistry (e.g. disulfide bond) would be introduced onto the polymer backbonefor drug conjugation. Polyethylene glycol would be added to the polymers to increase their biocompatibility and bioavailability.(2) Characterization of polymersThe structures, molecular weights and compositions of the synthesized peptide polymers will be characterized by NMR, mass spectrometry, GPCand HPLCetc. Their physicochemical properties tested by UV-visible spectroscopy, fluorescence spectroscopy, dynamic light scattering, zeta potential analysis and electron microscopy, and their membrane disruptive activity tested in a well established haemolysis model system.(3) Testing of biological payloads in the model cell systemsiRNA, therapeutic peptide and antibody payloads against Bcl-2 would be tested in the model cellular system for their biological potency. There are known model systemsin which Bcl-2 antagonists are active, such as in Jurkat or HL-60 cells, where the assays for apoptosis and cell proliferation are well established. For this stage of testing, the siRNA payload would be delivered by standard cationic lipid transfection, while the peptide and antibody would be encoded on expression plasmids, delivered via lentiviral transfection.(4) Combination of polymer delivery and biological payloadsBiological payloads such as siRNA, therapeutic peptide and antibody would be directly conjugated onto the polymer delivery system through cleavable linkage. siRNA payload could also be complexed with the stimuli-responsive anionic polymers via the use of cationic packaging modules (e.g. cationic polymers, lipids or ions). At this stage different chemistries could be explored and the combination efficiency and reproducibility assessedby biophysical analysis. Control reagents will also be prepared, e.g. using existing delivery technology systems such as TAT peptides, for later comparison.(5) Testing of polymer-payload conjugates/complexes in the model cell systemIn the model cell system the polymer-payload entities would be compared for their biological potency in dose titration and time course studies to compare the different payloads. Confocal microscopy would be used to study the mechanisms and kinetics of cell uptake and to visualise the distribution of polymers and payloads within the cell compartments. Flow cytometry would be used for quantitative analysis of cell uptake.(6) Biomembrane activity and cytotoxicityThe distinct but closely related research is to understand the activity of nano-systems at the biological membrane level. Dr Rongjun Chen has established production collaborations with Prof Nelson (Centre for Molecular Nanoscince/School of Chemistry, University of Leeds) on interaction of polymers with electrochemical lipid model membrane systems. Cytotoxicity of the polymers and/or polymer-payload entities towards model cell lines will be tested by MTT assay for metabolic activity, propidium iodide assay for membrane integrity, TEM for morphology of intracellular compartments and Comet assay for DNA damage.(7) Extending the polymer systemWith the model delivery system established, the aim is to optimise delivery to both cytoplasm and nucleus, in particular optimising endosomal release through careful study of the fate of polymers with different chemical modifications by confocal microscopy. There are further opportunities to optimise the biodegradation of the polymer through chemical changes as well as to look within different cellular systems and to look at other intracellular and nuclear targets.F. Schedule of researchPeriod of Study: 36 months from 1st October, 2011Programme: Full-timeMaximum time limit for submission of PhD thesis 30th September, 2015.The detailed schedule for different research stages shown in the above Section E is shown in the Table 1 Work plan.G. Introduction of work in China after graduationAfter obtaining my PhD degree from University of Leeds, I will return to Beijing University of Chemical Technology starting my academic career in the Department of Biomaterials of the College of Materials Science and Engineering. I will maintain my collaborative network including my PhD supervisor Dr Rongjun Chen in Leeds and other scientists/engineers/clinicians within and outside of the University Leeds. I will devote myself in the research area of drug delivery and biological therapy for various diseases including cancers. This area of research in nanomedicine aligns well with the research strategies of Beijing University of Chemical Technology, and will also have considerable potential to make a very positive impact upon the development of biotechnology in China. After I return to China at the end of my studies, the multidisciplinary knowledge and skills at the interface of Chemistry, Biology and Medicine, and excellent scientific capabilities that I will have developed will make me succeed in my academic career to the great benefit of Chinese Biotechnology and develop new potential nanomedicinesto positively impact human health in China and worldwide.Specifically speaking, the PhD research will enable me to have a good understanding of the basic science surrounding drug delivery through a systematic assessmentof both the delivery component and the payload component in a general biological system. Current knowledge of intracellular drug delivery is not well-developed and this project aims to shed light on this area. This PhD project fits the concept of taking novel tools into biological systems to advance the fundamental understanding of complex biological processes and translate basic research into new and improved healthcare qualities and practice. It supports knowledge exchange between academia and industry. In addition, the area of research in nanomedicine within Beijing University of Chemical Technology would help catalyze/enhance cross-faculty interactions/collaborations with researchersin the areas of drug delivery, molecular imaging, polymer chemistry, drug design, nanoparticle synthesis and characterisation, computational modeling of membrane activity, nanotoxicology, physics, cell biology, and medicine, therefore facilitating translational research from basic sciences into healthcare initiatives.The projected total market for nanotechnology-enabled drug delivery is predicted to rise to $26 billion by 2012. The area of research in drug delivery and therapy is of strategic interest to biopharmaceutical and healthcare sectors as it would enable new opportunities to make potent and specific drugs to intracellular targets which are currently inaccessible with existing technologies. The key knowledge and research skills developed from my PhD project will be of interest to all nanotechnology, drug delivery and medical scientists anxious to generate much superior disease treatments leading to substantial savings in healthcare budget, better health and improved quality of life.Table 1. Work planTASK Oct 2011–Sep 2012 Oct 2012–Sep 2013 Oct 2013–Sep 2014 Oct 2014–Sep 2015Q1 Q2 Q3 Q4 Q1 Q2 Q3 Q4 Q1 Q1 Q3 Q4 Q1 Q2 Q3 Q4(1) Polymer synthesis(2) Characterization of polymers(3) Testing of biological payloadsin the model cell system(4) Combination of polymerdelivery and biological payloads(5) Testing of polymer-payloadconjugates/complexes in themodel cell system(6) Biomembrane activity andcytotoxicity(7) Extending the polymer system(8) Writing up PhD thesisApproval by PhD supervisorSignatureName Dr Rongjun ChenPosition Group Leader of Biomaterials and Drug Delivery Group, BHRC Senior Translational Research Fellow,Centre for Molecular Nanoscience, School of Chemistry, University of Leeds, UKTime 12 March 2011Page of 778。
Biomedical Materials and Engineering
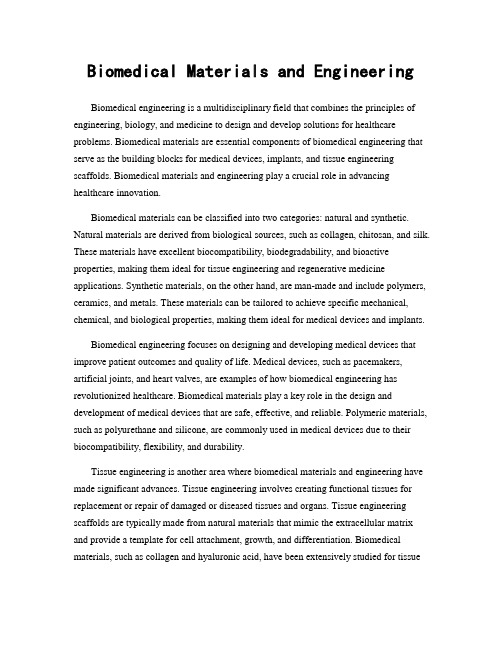
Biomedical Materials and EngineeringBiomedical engineering is a multidisciplinary field that combines the principles of engineering, biology, and medicine to design and develop solutions for healthcare problems. Biomedical materials are essential components of biomedical engineering that serve as the building blocks for medical devices, implants, and tissue engineering scaffolds. Biomedical materials and engineering play a crucial role in advancing healthcare innovation.Biomedical materials can be classified into two categories: natural and synthetic. Natural materials are derived from biological sources, such as collagen, chitosan, and silk. These materials have excellent biocompatibility, biodegradability, and bioactive properties, making them ideal for tissue engineering and regenerative medicine applications. Synthetic materials, on the other hand, are man-made and include polymers, ceramics, and metals. These materials can be tailored to achieve specific mechanical, chemical, and biological properties, making them ideal for medical devices and implants.Biomedical engineering focuses on designing and developing medical devices that improve patient outcomes and quality of life. Medical devices, such as pacemakers, artificial joints, and heart valves, are examples of how biomedical engineering has revolutionized healthcare. Biomedical materials play a key role in the design and development of medical devices that are safe, effective, and reliable. Polymeric materials, such as polyurethane and silicone, are commonly used in medical devices due to their biocompatibility, flexibility, and durability.Tissue engineering is another area where biomedical materials and engineering have made significant advances. Tissue engineering involves creating functional tissues for replacement or repair of damaged or diseased tissues and organs. Tissue engineering scaffolds are typically made from natural materials that mimic the extracellular matrix and provide a template for cell attachment, growth, and differentiation. Biomedical materials, such as collagen and hyaluronic acid, have been extensively studied for tissueengineering applications and have shown promising results in regenerating tissues such as skin, bone, and cartilage.Nanotechnology is a rapidly growing area of biomedical engineering that has enormous potential for improving healthcare. Nanoparticles, nanotubes, and nanofibers offer unique properties that can be tailored for various biomedical applications. For example, nanoparticles can be used as drug carriers to deliver drugs to specific targets in the body, while nanofibers can be used as tissue engineering scaffolds. Biomedical materials made from nanotechnology have the potential to revolutionize drug delivery, cancer treatment, and regenerative medicine.Biomaterials play a critical role in ensuring the safety and efficacy of medical devices and implants. Before a medical device or implant is approved for use, it undergoes rigorous testing to ensure that it meets safety and efficacy standards. Biomedical materials are tested for biocompatibility, degradation, mechanical properties, and durability. In addition, regulatory agencies such as the U.S. Food and Drug Administration (FDA) provide guidelines for the development, testing, and approval of medical devices and implants.In conclusion, biomedical materials and engineering have made significant contributions to healthcare innovation. Biomedical materials are essential components of medical devices, tissue engineering scaffolds, and regenerative medicine. Biomedical engineering has revolutionized healthcare by designing and developing medical devices that improve patient outcomes and quality of life. Advances in nanotechnology have enormous potential for improving drug delivery, cancer treatment, and regenerative medicine. Biomedical materials and engineering play a crucial role in ensuring the safety and efficacy of medical devices and implants. It is an exciting time for biomedical engineering and materials science, and the possibilities for future innovation are endless.。
交联PMMA@玻尿酸微胶囊的制备及其释放性能

交联PMMA@玻尿酸微胶囊的制备及其释放性能王建平,王凡,王喜睿,王学晨,李伟,张兴祥(天津工业大学天津市先进纤维与储能技术重点实验室,天津300387)摘要:为成功制备玻尿酸微胶囊,在水/油(W/O )反相乳液体系中通过自由基聚合制备了以玻尿酸水溶液为芯材、季戊四醇四丙烯酸酯(PET4A )交联甲基丙烯酸甲酯(MMA )聚合物为壁材的微胶囊。
采用扫描电子显微镜(SEM )、透射电子显微镜(TEM )、傅里叶红外光谱仪(FTIR )、激光散射粒度分布分析仪(LSPSDA )、紫外分光光度计(UV )对微胶囊的形貌、结构、粒径分布、包覆率及释放性能进行了表征。
结果表明:微胶囊具有不规则球形及核壳结构形貌。
当油水比为10颐1、芯壁比为1颐1、玻尿酸用量为1.5mg/mL 、交联剂添加量为0.67%(相对于壁材)时,微胶囊包覆率与载药率分别达到极大值81.40%和1.221%,表面形貌最好,其粒径在1.4~2.0滋m 之间。
微胶囊累计释放量随交联剂用量的升高而下降,同时微胶囊具有pH 响应性,pH =5.5时累计释放量最大。
关键词:W/O 反相乳液;玻尿酸;季戊四醇四丙烯酸酯;甲基丙烯酸甲酯;微胶囊;释放性能;自由基聚合中图分类号:TB34文献标志码:A文章编号:员远苑员原园圆源载(圆园23)园6原园园17原06Preparation and release properties of crosslinked PMMA@hyaluronic acid microcapsulesWANG Jianping ,WANG Fan ,WANG Xirui ,WANG Xuechen ,LI Wei ,ZHANG Xingxiang(Tianjin Key Laboratory of Advanced Fibers and Energy Storage Technology ,Tiangong University ,Tianjin 300387,China )Abstract :In order to prepare hyaluronic acid microcopsule袁microcapsules with hyaluronic acid aqueous solution as thecore and pentaerythritol tetraacrylate 渊PET4A冤cross-linked methyl methacrylate 渊MMA冤polymer as the wall were prepared by a free radical polymerization in a W/O inverse emulsion system.The morphology袁structure袁particle size distribution袁coating ratio and release property of the microcapsules were characterized by scanning electron microscopy 渊SEM冤袁transmission electron microscopy 渊TEM冤袁Fourier transform infrared spectroscopy渊FTIR冤袁laser scattering particle size distribution analyzer 渊LSPSDA冤and ultraviolet spectrophotometer 渊UV冤.The results showed that the microcapsules had irregular spherical shape and core-shell structure.When the oil/water ratio was 10颐1袁the core/wall ratio was 1颐1袁the dosage of hyaluronic acid was 1.5mg/mL袁and the addition of crosslinking agent was 0.67%渊relative to the wall material冤袁the encapsulation efficiency and drug loading rate of the microcapsules reached the maximum of 81.40%and 1.221%袁respectively.The surface morphology was the best袁and the particle size was within 1.4-2.0滋m.The cumulative release of microcapsules decreased withthe increase of the amount of crosslinking agent.At the same time袁the microcapsules were pH-responsive袁andthe cumulative release was the most when pH =5.5.Key words :W/O inverse emulsion曰hyaluronic acid曰pentaerythritol tetraacrylate曰methyl methacrylate曰microcapsule曰re鄄lease property曰free radical polymerization玻尿酸学名“透明质酸”,是一种天然安全、可降解的生物材料,其质地粘稠透明像玻璃一样,因此命名为“hyaluronic acid ”,简称为“HA ”[1]。
学术论文的语言表达(英文)
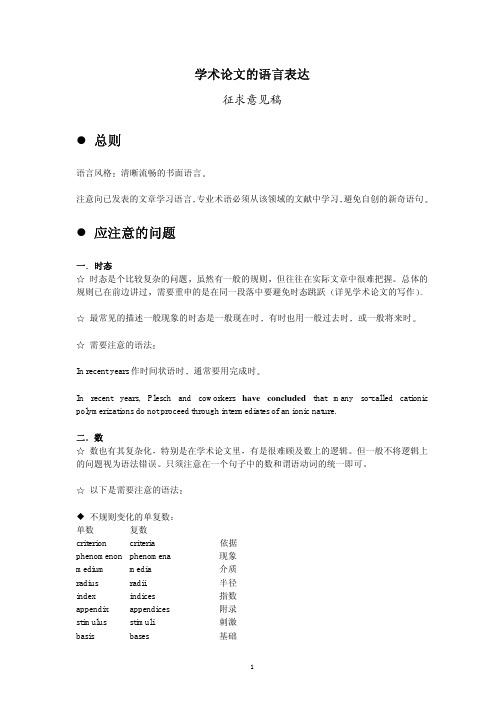
Much research has been done to redirect the tropism of retroviruses by engineering the envelope glycoprotein.
Most research on poly(ortho esters) has focused on the synthesis of polymers.
一些数有特殊性的名词 data, kinetics 通常作复数名词
research, work: 不可数名词
The data in Table 1 are obtained from the reactions at different temperatures.
The kinetics of polymerization within each droplet are the same as those for the corresponding bulk polymerization.
The use of IPNs has received a great deal of attention in recent years and different kinds of IPN have been synthesized with different compositions.
天然温敏性高分子药物载体材料

天然温敏性高分子药物载体材料摘要:对环境刺激响应的刺激性响应材料在药物运输方面有重要的作用。
本文旨在介绍几种天然温敏性高分子在药物运输方面的应用,并对此天然高分子与合成高分子在药物运输中的优缺点。
关键词:天然高分子药物载体温敏性、八、、》刖言刺激性响应材料因其对环境有特殊的响应而得到人们的关注,随着近几年医用高分子材料的发展,人们已可以根据特定的生理需求来制造药物载体⑴。
刺激性响应高分子也叫智能高分子、环境响应高分子,当外界环境发生微小变化时,它们能迅速地发生相应的物理化学变化,根据响应因素的不同,可以分为光响应高分子、超声响应高分子、PH响应高分子、温度响应高分子等等。
这些高分子在外界因素发生改变时,它们可能发生疏水/亲水转变、构象转变、溶解度改变、胶束化等等⑵。
因此可以将高分子做为药物载体,从而对药物释放进行有效的控制。
这这些刺激性响应材料中,温敏性高分子是研究最广泛的,所以本文将重点介绍几种温敏性天然高分子在药物载体上的应用(见表1)。
Table 1, A list of natural and synthetic thermoresponsive homopolymers and copolymers with their corresponding thermal phase transition temperatures (cont.).Study (ytar}Name Abbreviation LCST/UCST mRef-Natural polymers and denvativesChenite er 訓(2000} a ndMolinaro efat (2002)C hitosan-glyGerophosphste ChiTQMn-GP-37加削]Persson et at. (2000)Methyicelluiose MC50阿Schild (1990}Hydroxypropylcellu low MPC42J91 Persson et刖(2000)Ethyl (hydroxyethyl}cell ulnse EHEC S5Miyazaki (1998)'X/loglucan (with 44% removal of galactose)22-27【制Chllkoti 就 at (2006)Elastin-lik& polypeptides ELP0-100阿]Ge et at, (2010)ELP^A^-gO]的【叫Meyer^t^/. (2001)ELPIVgA^ISO]do 1«]ELP [V^Gj-160]5SUrryeta/. (1991)ft)ly(VPGVG)27[H&] 'Fturcinic nomencbture: The first letter in theccfx^ymer name indicates ifhat the ph愕k制of the starting polymer 海迢liquid (1). The Laft number the weight cantenrt of the PEO block <an terms of weight percent), whib the remaining numbers give an indkatian af the mDtecularwietght of the PPO blcxk (taken from) [102]. Adapted from |IU],温敏性高分子是在微观上分子随着温度发生微小改变,从而达到宏观上材料性能的.温敏性高分子在控制药物释放上的应用改变的一类材料。
高分子英语课文翻译
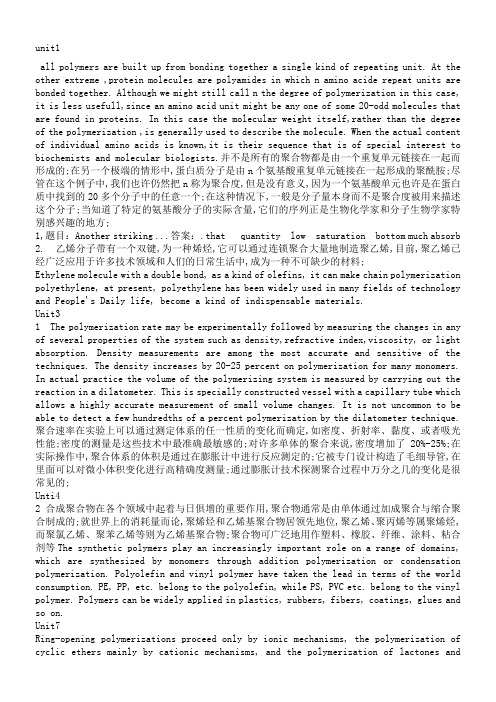
unit1all polymers are built up from bonding together a single kind of repeating unit. At the other extreme ,protein molecules are polyamides in which n amino acide repeat units are bonded together. Although we might still call n the degree of polymerization in this case, it is less usefull,since an amino acid unit might be any one of some 20-odd molecules that are found in proteins. In this case the molecular weight itself,rather than the degree of the polymerization ,is generally used to describe the molecule. When the actual content of individual amino acids is known,it is their sequence that is of special interest to biochemists and molecular biologists.并不是所有的聚合物都是由一个重复单元链接在一起而形成的;在另一个极端的情形中,蛋白质分子是由n个氨基酸重复单元链接在一起形成的聚酰胺;尽管在这个例子中,我们也许仍然把n称为聚合度,但是没有意义,因为一个氨基酸单元也许是在蛋白质中找到的20多个分子中的任意一个;在这种情况下,一般是分子量本身而不是聚合度被用来描述这个分子;当知道了特定的氨基酸分子的实际含量,它们的序列正是生物化学家和分子生物学家特别感兴趣的地方;1,题目:Another striking ...答案:.that quantity low saturation bottom much absorb 2. 乙烯分子带有一个双键,为一种烯烃,它可以通过连锁聚合大量地制造聚乙烯,目前,聚乙烯已经广泛应用于许多技术领域和人们的日常生活中,成为一种不可缺少的材料;Ethylene molecule with a double bond, as a kind of olefins, it can make chain polymerization polyethylene, at present, polyethylene has been widely used in many fields of technology and People's Daily life, become a kind of indispensable materials.Unit31 The polymerization rate may be experimentally followed by measuring the changes in any of several properties of the system such as density,refractive index,viscosity, or light absorption. Density measurements are among the most accurate and sensitive of the techniques. The density increases by 20-25 percent on polymerization for many monomers. In actual practice the volume of the polymerizing system is measured by carrying out the reaction in a dilatometer. This is specially constructed vessel with a capillary tube which allows a highly accurate measurement of small volume changes. It is not uncommon to be able to detect a few hundredths of a percent polymerization by the dilatometer technique. 聚合速率在实验上可以通过测定体系的任一性质的变化而确定,如密度、折射率、黏度、或者吸光性能;密度的测量是这些技术中最准确最敏感的;对许多单体的聚合来说,密度增加了20%-25%;在实际操作中,聚合体系的体积是通过在膨胀计中进行反应测定的;它被专门设计构造了毛细导管,在里面可以对微小体积变化进行高精确度测量;通过膨胀计技术探测聚合过程中万分之几的变化是很常见的;Unti42 合成聚合物在各个领域中起着与日俱增的重要作用,聚合物通常是由单体通过加成聚合与缩合聚合制成的;就世界上的消耗量而论,聚烯烃和乙烯基聚合物居领先地位,聚乙烯、聚丙烯等属聚烯烃,而聚氯乙烯、聚苯乙烯等则为乙烯基聚合物;聚合物可广泛地用作塑料、橡胶、纤维、涂料、粘合剂等The synthetic polymers play an increasingly important role on a range of domains, which are synthesized by monomers through addition polymerization or condensation polymerization. Polyolefin and vinyl polymer have taken the lead in terms of the world consumption. PE, PP, etc. belong to the polyolefin, while PS, PVC etc. belong to the vinyl polymer. Polymers can be widely applied in plastics, rubbers, fibers, coatings, glues and so on.Unit7Ring-opening polymerizations proceed only by ionic mechanisms, the polymerization of cyclic ethers mainly by cationic mechanisms, and the polymerization of lactones andlactones by either a cationic or anionic mechanism. Important initiators for cyclic ethers and lactone polymerization are those derived from aluminum alkyl and zinc alkyl/water systems. It should be pointed out that substitution near the reactive group of the monomer is essential for the individual mechanism that operates effectively in specific cases; for example, epoxides polymerize readily with cationic and anionic initiators, while fluorocarbon epoxides polymerize exclusively by anionic mechanisms.开环聚合反应只能通过离子机理进行,环醚的开环聚合主要通过阳离子机理,而内酯和内酰胺的聚合物是通过阳离子或阴离子机理;对于环醚和内酯型聚合物很重要的引发剂是那些来自于烷基铝和烷基锌/水的体系;应该指出的是对于在活性基团附近有取代的单体,只能由单一机理,这一机理是在特定条件下的有效;1 Polymers can be classified into two main groups, addition polymers and ___condensation__ polymers. This classification is based on whether or not the repeating unit of the polymer contains the same atoms __as____ the monomer. The repeating unit of an addition polymer is identical _with/to____ the monomer, while condensation polymers contain __different/less___ because of formation of __compound/byproduct___ during the polymerization process. The corresponding polymerization processed would then be called addition polymerization and condensation polymerization. As was mentioned earlier, this classification can result ___in__ confusion, since it has been shown in later years that many important types of polymers can be _prepared by both addition and condensation processes. For example, polyesters, polyamides and polyurethanes are usually considered to be _condensation____ polymers, but they can be prepared by addition as well as by condensation reaction. Similarly, polyethylene normally considered an _addition_ polymer, can also be prepared by _condensation_ reaction.2. Answer the following questions in English1 What is chain polymerization Manyolefinicandvinylunsaturatedcompoundsareabletoformchain-likemacromoleculesthrougheliminationofdoublebond.2 Which kinds of monomers can carry out step-growth polymerization processThere are two kinds of monomers could carry out step-growth polymerization process. One ispolyfunctionalmonomers and the other isasinglemonomercontainingbothtypesoffunctional groups.3 What properties of polymers can be based on for measuring the molecular weightThe molecular weight of polymer could be measured based on colligativeproperties, lightscattering, viscosity, ultracentrifugation sedimentation.3. Please write out at least 10 kinds of polymers both in English and in Chinesethe corresponging chemical structure5 In general,head-to-tail addition is considered to be the predominant mode of propagation in all polymerizations;However,when the substitutes on the monomer are small and do not offer appreciable steric hindrance to the approaching radical or do not have a large resonance stabilizing effect,as in the case of fluorine atoms,sizable amounts of head-to-head propagation may occur. The effect of increasing polymerization temperature is to increase the amount of head-to-head placement;Increased temperature leads to less selective more random propagation but the effect is not large. Thus,the head-to-head content in poly vinyl acetate only increases from to percent when the polymerization temperature in increased from 30 to 90 ℃.通常在所有聚合物的链增长中,头-尾加成是主要方式;然而,当单体中的取代基很小对接近的自由基没有空间阻碍或没有较大的共振稳定作用,如氟原子,则有相当量的头头增长发生;提高聚合温度的影响是提高头-头排列的量;温度的提高导致较少的选择更多的无规增长,但影响不大;因而,在聚乙酸乙烯酯中,当聚合温度由30C提高到90C,头-头含量仅由%提高到%;2.Write out an abstract in English for the text in this unitPolymers with different structures present various properties. Usually, polymers are divided into three categories, . plastic, elastomer, fiber with different initial modulus range respectively. Polymers show quite different behaviors due to the different interchain forces in elastomer and fiber. However, with the advent of new techniques and mechanisms to improve the structure of polymers, polymers may be classified and named according to the mechanism, and their properties will largely depend on the structure. 3.Put the following words into Chineseentanglement 纠缠 irregularity 无规 sodium isopropylate异丙醇钠 permeability渗透性crystallite 微晶stoichiomertric balance 当量平衡fractionation分馏法light scattering光散射 matrix 基体 diffraction衍射4.Put the following words into English形态 morphology 酯化 esterification 异氰酸酯isocyanate杂质impurity 二元胺 diamine 转化率change ratio 多分散性polydispersity 力学性能mechanical property 构象conformation 红外光谱法infrared spectroscopy常见聚合物命名1常见杂链和元素有机聚合物类型Polyamide ----聚酰胺. Polyester----聚酯 Poly‘urethane ------聚氨酯 Polysiloxane -------聚硅氧烷Phenol-formaldehyde----酚醛.Urea-formaldehyde-----脲醛Polyureas------聚脲 Polysulfide -----聚硫Polyacetal-------聚缩醛 Polysulfone polysulphone------聚砜 Polyether---------聚醚第五单元Traditional methods of living polymerization are based on ionic, coordination or group transfer mechanisms.活性聚合的传统方法是基于离子,配位或基团转移机理;Ideally, the mechanism of living polymerization involves only initiation and propagation steps.理论上活性聚合的机理只包括引发和增长反应步骤;All chains are initiated at the commencement of polymerization and propagation continues until all monomer is consumed.在聚合反应初期所有的链都被引发,然后增长反应继续下去直到所有的单体都被消耗殆尽;A type of novel techniques for living polymerization, known as living possibly use “controlled” or “mediated” radical polymerization, is developed recently. 最近开发了一种叫做活性自由基聚合的活性聚合新技术;The first demonstration of living radical polymerization and the current definition of the processes can be attributed to Szwarc.第一个活性自由基聚合的证实及目前对这一过程的解释或定义,应该归功于Szwarc;Up to now, several living radical polymerization processes, including atom transfer radical polymerization ATRP, reversible addition-fragmentation chain transfer polymerization RAFT, nitroxide-mediated polymerization NMP, etc., have been reported one after another.到目前为止,一些活性自由基聚合过程,包括原子转移自由基聚合,可逆加成-断裂链转移聚合,硝基氧介导聚合等聚合过程一个接一个被报道;The mechanism of living radical polymerization is quite different not only from that of common radical polymerization but also from that of traditional living polymerization. 活性自由基聚合的机理不仅完全不同于普通自由基聚合机理,也不同于传统的活性聚合机理;It relies on the introduction of a reagent that undergoes reversible termination with the propagating radicals thereby converting them to a following dormant form:活性自由基聚合依赖于向体系中引入一种可以和增长自由基进行可逆终止的试剂,形成休眠种:The specificity in the reversible initiation-termination step is of critical importance in achieving living characteristics.这种特殊的可逆引发-终止反应对于获得分子链活性来说具有决定性的重要意义;This enables the active species concentration to be controlled and thus allows such a condition to be chosen that all chains are able to grow at a similar rate if not simultaneously throughout the polymrization.可逆引发终止使活性中心的浓度能够得以控制;这样就可以来选择适宜的反应条件,使得在整个聚合反应过程中只要没有平行反应所有的分子链都能够以相同的速度增长;This has, in turn, enabled the synthesis of polymers with controlled composition, architecture and molecular weight distribution.这样就可以合成具有可控组成,结构和分子量分布的聚合物;They also provide routes to narrow dispersity end-functional polymers, to high purity block copolymers, and to stars and other more complex architecture.这些还可以提供获得狭窄分布末端功能化聚合物,高纯嵌段共聚物,星型及更复杂结构高分子的合成方法;The first step towards living radical polymerization was taken by Ostu and his colleagues in 1982.活性自由基聚合是Ostu和他的同事于1982年率先开展的;In 1985, this was taken one step further with the development by Solomon et al. of nitroxide-mediated polymerization NMP.1985年,Solomon等对氮氧化物稳定自由基聚合的研究使活性自由基聚合进一步发展;This work was first reported in the patent literature and in conference papers but was not widely recognized until 1993 when Georges et al. applied the method in the synthesis of narrow polydispersity polystyrene.这种方法首先在专利文献和会议论文中报道,但是直到1993年Georges等把这种方法应用在窄分子量分布聚苯乙烯之后,才得以广泛认知;The scope of NMP has been greatly expended and new, more versatile, methods have appeared. NMP的领域已经得到很大的延展,出现了新的更多样化的方法;The most notable methods are atom transfer radical polymerization ATRP and polymerization with reversible addition fragmentation RAFT.最引人注目的方法是原子转移自由基聚合和可逆加成断裂聚合;到2000年,这个领域的论文已经占所有自由基聚合领域论文的三分之一;如图所示;Naturally, the rapid growth of the number of the papers in the field since 1995 ought to be almost totally attributable to development in this area. 、自然地,纸的数量的迅速增长在领域,因为1995在这个区域应该是几乎完全可归属的到发展;UNIT9 Structure and Properties of Polymers 聚合物的结构和性质Most conveniently, polymers are generally subdivided in three categories, namelyviz., plastics, rubbers and fibers. 很方便地,聚合物一般细分为三种类型,就是塑料,橡胶和纤维; In terms of initial elastic modules, rubbers ranging generally between 106 to 107dynes/cm2, represent the lower end of the scale, while fibers with high initial modjulai, of 1010 to 1011dynes/cm2 are situated on the upper end of the scale; plastics, having generally an initial elastic modulus of 108 to 109dynes/cm2, lie in-between. 就初始弹性模量而言,橡胶一般在 6到107达因平方厘米,在尺度的低端, 10到1011达因平方厘米,尺度的高端,而纤维具有高的初始模量, 达到10到1011达因平方厘米,尺度的高端,塑料的弹性模量一般在 8到109达因平方厘米,在尺度的中间As is found in all phases of polymer chemistry, there are many exceptions to this categorization. 正如高分子化学的各个部分都可以看到的那样,在高分子化学的所有阶段,我们都可以发现,这种分类方法有许多例外的情况;An elastomer or rubber results from a polymer having relatively weak interchain forces and high molecular weights. 弹性体是具有相对弱的链之间作用力和高分子量的聚合物; When the molecular chains are “straightened out” or stretched by a process of extension, they do not have sufficient attraction for each other to maintain the oriented state and will retract once the force is released. This is the basis of elastic behavior. 当通过一个拉伸过程将分子链拉直的时候,分子链彼此之间没有足够的相互吸引力来保持其取向状态,作用力一旦解除,将发生收缩;这是弹性行为的基础;However, if the interchain forces are very great, a polymer will make a good fiber. 然而,如果分子链之间的力非常大,聚合物可以用做纤维;Therefore, when the polymer is highly stretched, the oriented chain will come under the influence of the powerful attractive forces and will “crystallize” permanently in a more or less oriented matrix. 因此,当聚合物被高度拉直的时候,取向分子链在不同程度取向的母体中将受强引力的影响而“永久地结晶;These crystallization forces will then act virtually as crosslinks, resulting in a material of high tensile strength and high initial modulus, ., a fiber. 而后,这些结晶力实际上以交联方式作用,产生高拉伸强度和高初始模量的材料,如纤维;Therefore, a potential fiber polymer will not become a fiber unless subjected to a “drawing” process, ., a process resulting in a high degree of intermolecular orientation. 因此,一个可能的潜在的纤维高分子不会变成纤维,除非经历一个拉伸过程, 即, 这导致分子间高度取向的拉伸过程;Crosslinked species are found in all three categories and the process of crosslinking may change the cited characteristics of the categories. 交联的种类在所有三种类型塑料,橡胶,纤维中找到,而交联过程可以改变分类的引用特征;Thus, plastics are known to possesspzes a marked range of deformability in the order of 100 to 200%; they do not exhibit this property when crosslinked, however. 因此,我们熟知塑料具有的形变能力大约在100-200%范围内,然而当交联发生时塑料不能展示这个性能; Rubber, on vulcanization, changes its properties from low modulus, low tensile strength, low hardness, and high elongation to high modulus, high tensile strength, high hardness, and low elongation. 对橡胶而言,硫化可以改变其性质,从低模量,低拉伸强度,低硬度及高拉伸率到高模量,高拉伸强度,高硬度及低拉伸率;Thus, polymers may be classified as noncrosslinked and crosslinked, and this definition agrees generally with the subclassification in thermoplastic and thermoset polymers. 这样,聚合物可以分为非交联和交联的,这个定义与把聚合物细分为热塑性和热固性聚合物相一致; From the mechanistic point of view, however, polymers are properly divided into addition polymers and condensation polymers. Both of these species are found in rubbers, plastics, and fibers. 然而,从反应机理的观点看,聚合物可以分成加聚物和缩聚物;这些种类聚合物在塑料,橡胶和纤维中都可以找得到;In many cases polymers are considered from the mechanistic point of view. Also, the polymer will be named according to its source whenever it is derived from a specific hypothetical monomer, or when it is derived from two or more components which are built randomly into the polymer. 在许多情况下,聚合物可以从反应机理的角度考虑分类; 每当聚合物来自于一个假象单体,或来自于两个或两个以上组成物无规则构建聚合物时,也可以根据聚合物的来源来命名; This classification agrees well with the presently used general practice. 这种分类方法与目前实际情况相符合;When the repeating unit is composed of several monomeric components following each other in a regular fashion, the polymer is commonly named according to its structure. 当重复单元由几个单体组成物规则排布,聚合物通常根据它的结构来命名;It must be borne in mind that, with the advent of Ziegler-Natta mechanisms and new techniques to improve and extend crystallinity, and the closeness of packing of chains, many older data given should be critically considered in relation to the stereoregular and crystalline structure. 必须记住,随着Ziegler-Natta机理,以及提高结晶度和链堆砌紧密度新技术的出现,对许多过去已经得到的关于空间结构和晶体结构旧的资料,应当批判地接受;The properties of polymers are largely dependent on the type and extent of both stereoregularity and crystallinity. As an example, the densities and melting points of atactic and isotactic species are presented in Table . 聚合物的性质主要依靠立体规整性和结晶度的类型和程度;如,无规立构和全同立构物质的密度和熔点展示在表中 ;UNIT11 Functional PolymersFunctional polymers are macromolecules to which chemically functional groups are attached; they have the potential advantages of small molecules with the same functional groups. 功能聚合物是具有化学功能基团的大分子,这些聚合物与具有功能聚合物是具有化学功能基团的大分子, 相同功能基团的小分子一样具有潜在的优点;Their usefulness is related both to the functional groups and to the nature of the polymers whose characteristic properties depend mainly on the extraordinarily large size of the molecules.它们的实用性不仅与功能基团有关,而且与巨大分子尺寸带来的聚合物特性有关;The attachment of functional groups to a polymer is frequently the first step towards the preparation of a functional polymer for a specific use. 把功能基团连接到聚合物上常常是制备特殊用途功能高分子的第一步;However, the proper choice of the polymer is an important factor for successful application. 然而,对成功应用而言,选择适当的聚合物是的一个重要因素;In addition to the synthetic aliphatic and aromatic polymers, a wide range of natural polymers have also been functionalized and used as reactive materials. 除了合成的脂肪组和芳香组聚合物之外,许多天然高分子也被功能化,被用做反应性材料;Inorganic polymers have also been modified with reactive functional groups and used in processes requiring severesi’vi service conditions. 无机聚合物也已经用反应功能基团改性,被用于要求耐用条件的场合;In principle, the active groups may be part of the polymer backbone or linked to a side chain as a pendant group either directly or viavai a space rs’peis group. 理论上讲,活性基团可以是聚合物主链上的一部分,或者直接连接到侧链或通过一个中间基团的侧基;A required active functional group can be introduced onto a polymeric support chain 1 by incorporation during the synthesis of the support itself through polymerization or copolymerization of monomers containing the desired functional groups, 2 by chemical modification of a nonfunctionalized performed support matrix and 3 by a combination of 1 and 2. 所需的活性功能基团可以通过几种方法引入到聚合物主链上, 1在主链的合成过程中,通过聚合或共聚合含有理想功能基团的单体来获得,2通过对已有的非功能化主链进行化学改性的方法,3通过结合1和2来获得;Each of the two approaches has its own advantages and disadvantages, and one approach may be preferred for the preparation of a particular functional polymer when the other would be totally impractical.两种途径中的每一种都有自身的优点和缺点,对特殊功能聚合物的制备而言,当其他方法都无法实现时,所选的方法或许是更合适的;The choice between the two ways to the synthesis of functionalized polymers depends mainly on the required chemical and physical properties of the support for a specific application. 功能聚合物合成的两种方法中,如何选择主要取决于特殊应用要求的主链聚合物的化学和物理性质;Usually the requirements of the individual system must be thoroughly examined in order to take full advantage of each of the preparative techniques. 为了充分利用每种制备方法,必须全面地考察独立体系的要求;Rapid progress in the utilization of functionalized polymeric materials has been noted in the recent past. 近年来,功能化聚合物材料的使用方面有了飞速的发展;Interest in the field is being enhanced due to the possibility of creating systems that combine the unique properties of conventional active moieties and those of high molecular weight polymers. 由于能够制造出来兼有活性官能团特性和高分子量聚合物性能的功能聚合物,所以,人们对功能聚合物这个领域的兴趣与日俱增;The successful utilization of these polymers are based on the physical form, solution behavior, porosity, chemical reactivity and stability of the polymers. 这些聚合物的成功利用,基于功能聚合物的物理形态,溶液行为,空隙率,化学活性及稳定性;The various types of functionalized polymers cover a broad range of chemical applications, including the polymeric reactants, catalysts, carriers, surfactants, stabilizers,ionexchange resins, etc.各种功能化聚合物类型覆盖化学应用的宽广领域,包括聚合物试剂,催化剂, 载体,表面活性剂,稳定剂,离子交换树脂等;In a variety of biological and biomedical fields, such as the pharmaceutical, agriculture, food industry and the like, they have become indispensable materials, especially in controlled release formulation of drugs and agrochemicals. 在生物学及生物医学领域中,如药物,农业,食品工业等, 在生物学及生物医学领域中,如药物,农业,食品工业等,功能聚合物是不可缺少的材料,尤其在药物和农药的控制释放配方上;Besides, these polymers are extensively used as the antioxidants, flame retardants, corrosion inhibitors, flocculating agents, antistatic agents and the other technological applications. 此外,这些聚合物被广泛地用做抗氧化剂,阻燃剂,缓蚀剂, 絮凝剂,抗静电剂及其他技术应用;In addition, the functional polymers possessp’zes broad application prospects in the high technology area as conductive materials, photosensitizers, nuclear track detectors, liquid crystals, the working substances for storage and conversion of solar energy, etc. 另外,功能化聚合物在高科技领域具有广阔的应用前景; 如导电材料,光敏剂,核径迹探测器,液晶,用于太阳能等的转化与储存的工作物质;第十二单元实验室制备氨基树脂氨基树脂是由氨基衍生物和醛在酸性或碱性条件下反应生产得到的其中最重要最具代表性的物质是脲醛树脂和蜜胺树脂; 药品:尿素,福尔马林37%,乙醇,2N NaOH, NaOH溶液,1N标准NaOH溶液,1N标准HCl溶液,冰醋酸,糠醇,三乙醇胺,木粉,磷酸钙,氯化铵, H2SO4溶液,Na 2SO3,1%乙醇百里酚酞指示剂溶液,三聚氰胺,甘油和单羟甲基脲; 装置:烧瓶和烧杯,500ml的三口烧瓶,加热套,机械搅拌器,冷凝器,迪安—斯达克塔分水器,烘箱,广泛试纸,试管,250mL的容量烧瓶,冰浴,10ml 的移液管,滴管,油浴和广口瓶; 酸性条件下制备脲醛树脂:为了证明尿素和甲醛在酸性条件下的迅速反应,将5 g尿素和6 mL福尔马林在试管中混合,振荡试管直到尿素全部溶解;滴加4滴 N H2SO4以调节溶液pH到4,观察析出沉淀所需要的时间,取出部分沉淀并比较此沉淀以及单羟甲基脲样品在水中的溶解性;制备脲醛树脂粘合剂:将600g1mole尿素和137g福尔马林放入500ml三口烧瓶中,并安装好机械搅拌器和回流冷凝器,通过用广泛试纸测定用2NNaOH溶液把混合物PH值调至7~~8,然后将混合物回流2小时;1每隔半小时用下面的方法测定一次混合物中的自由甲醛含量,直到水完全脱除为止;2 当混合物回流2小时后,将迪安—斯达克塔分水器安装在烧瓶和回流冷凝器之间 ;大约有40ml水被蒸馏,用5滴冰醋酸将溶液酸化;将44g糠醇和的三乙醇胺加入到反应混合液中,加热此溶液到90℃并恒温15分钟;将混合物冷却到室温;取出15g的树脂样品和由1g木粉,磷酸钙和氯化铵组成的硬化剂混合 ;将混合物进行室温固化;3将剩下的没有加工硬化剂的树脂放入广口瓶中并提交给实验导师;自由甲醛含量的测定:自由甲醛含量的测定:准备250mL 1N Na2SO3溶液,并中和该溶液,从而使其产生淡蓝色的百里酚酞指示剂溶液;在250ml锥形瓶中加入重为2到3克的树脂样品到100mL的水中,摇晃锥形瓶使锥形瓶内的溶液充分溶解;如果树脂不能溶解,加入乙醇可以帮助溶解;在冰浴中使溶液的温度下降到4℃,加25mL的1M Na2SO3溶液在100mL的烧瓶中,用移液管移取10ml标准的1N HCl溶液到烧瓶中,降温至4℃;加10-15滴百里酚酞指示剂溶剂到样品烧瓶中,调整溶液的颜色至淡蓝色;用冷水冷却以后迅速地转移酸式亚硫酸盐溶液到样品烧瓶中;4滴定溶液到百里酚酞的终点标准1N NaOH 溶液;CH2O+Na2SO3+H2O →CH2OHSO3-Na++NaOH通过中和树脂溶液的HCl溶液的量来测定自由甲醛的百分含量;三聚氰胺甲醛树脂的制备:在一个500ml的配置有机械搅拌器和一个冷凝器的反应器中加入63g 的三聚氰胺和122g的福尔马林37%;混合物回流40分钟;%自由甲醛需要每隔十分钟测定一次;自由甲醛的测定步骤如上所述;样品经过20分钟加热后,在烧瓶和冷凝器间插入一个迪安—斯达克分水器,从而有10mL的水被蒸馏掉;把未固化的样品放入螺丝帽的坛子中,连同固化的树脂一起交给实验指导老师;15单元到目前为止大多数的PVC生产通过悬浮聚合;在这个过程中,氯乙烯单体悬浮液体滴,在连续水相剧烈的搅拌和保护胶体的悬浮剂;使用单体溶自由基引发剂polymeri等自下而上发生在悬浮液滴内,通过一个机制,已被证明相当于本体聚合;商业植物是基于批量反应堆,这增加了支持的大小,多年来;原来的工厂建于1940年代通常由IOOO 加仑反应堆;在1960年代和1950年代这t0 3000一5000加仑和增加随后,在1970年代初,29000加仑反应堆系统开发的胫完全②,t0 44000加仑200立方米的德国公司Huls;目前一些新的工厂正在建造的反应堆由不到isooo加仑容量,有一个批处理大小约25吨单体;小型反应堆通常衬玻璃给光洁度,抵制存款的搁置在墙上;~大反应堆通常的抛光不锈钢;氯乙烯的聚合反应是一个放热反应的能力,移走热量通常试图减少反应时间的限制因素;随着规模的反应堆已经增加了表面积体积比,因此加重这一问题;内部冷却线圈通常不用作吸引存款和很难清洁,从而对产品性能有不利影响;问题通常是克服使用冷冻水或回流冷凝器的装置,通过氯乙烯单体的连续回流;利用其潜热冷却的目的;一个简单的悬浮聚合配方可能包含以下成分:冷水通常是首先向反应堆虽然有时预热;然后添加pH值调节剂紧随其后的是分散剂的形式解决方案;发起者年代立即撒到水相的表面密封反应堆然后撤离前去除氧,因为这可以增加聚合时间,影响产品性能;当引发反应完成乙烯氯化物被指控和加热反应堆的内容开始;反应但真正的,产品分子量的主要控制因素;通常是在50——70 'c导致反应堆压力范围100 - 165 psi;趋势是朝着大的操作只打开关闭反应堆维护或可能偶尔打扫道;”:在这种情况下所有的原料都是负责解决方案或分散体,一般不需要疏散的一步;当达到所需的转换了,通常75%一95%,反应可以如果需要化学short-stopped和剩余的大部分单体恢复;他产品泥浆然后剥下来非常低的残留氯乙烯治疗-水平表示“状态”姆温度升高,在反应堆或类似容器,或接触蒸汽在逆流多平台汽提塔;然后脱水离心法和由此产生的泥浆湿饼乾,多级闪蒸干燥机一般,虽然各种不同的干燥类型使用不同的生产;干燥后,产品是通过某种剥皮屏幕去除无关的大颗粒装袋之前或装载散装油轮;—T 16 Styrene-Butadiene Copolymer第十六单元丁二烯-苯乙烯共聚物合成橡胶工业,以自由基乳液过程为基础,在第二次世界大战期间几乎很快地形成;那时,丁苯橡胶制造的轮胎性能相当优越,使天然橡胶在市场黯然失色;丁苯橡胶的标准制法是组分重量分数组分重量分数丁二烯72 过硫酸钾苯乙烯25 肥皂片十二烷基硫醇水180 混合物在搅拌下50℃加热,每小时转化5%~6%,在转化率达70%~75%时通过加入“终止剂”聚合反应终止,例如对苯二酚大约的重量百分含量,抑制自由基并避免过量支化和微凝胶形成;未反应的丁二烯通过闪蒸去除,苯乙烯在萃取塔中通过蒸汽萃取剥离;在加入抗氧剂后,例如N-甲基-β-萘胺的重量百分含量,加入盐水,其次加入稀释的硫酸或硫酸铝后乳液凝胶;凝胶碎片被洗涤、干燥。
亮光色丁规格英文
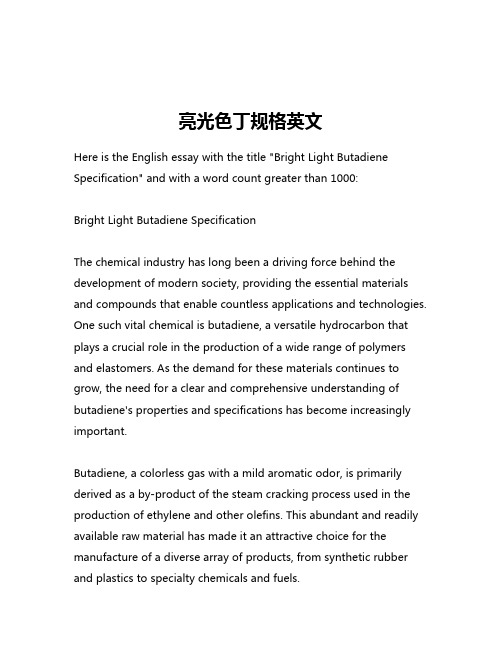
亮光色丁规格英文Here is the English essay with the title "Bright Light Butadiene Specification" and with a word count greater than 1000:Bright Light Butadiene SpecificationThe chemical industry has long been a driving force behind the development of modern society, providing the essential materials and compounds that enable countless applications and technologies. One such vital chemical is butadiene, a versatile hydrocarbon that plays a crucial role in the production of a wide range of polymers and elastomers. As the demand for these materials continues to grow, the need for a clear and comprehensive understanding of butadiene's properties and specifications has become increasingly important.Butadiene, a colorless gas with a mild aromatic odor, is primarily derived as a by-product of the steam cracking process used in the production of ethylene and other olefins. This abundant and readily available raw material has made it an attractive choice for the manufacture of a diverse array of products, from synthetic rubber and plastics to specialty chemicals and fuels.One of the key attributes of butadiene that has contributed to its widespread use is its ability to undergo polymerization, a process in which individual molecules are linked together to form long, chain-like structures. This property allows for the creation of a wide range of polymeric materials, each with its own unique set of physical and chemical characteristics.In the context of the bright light butadiene specification, the focus is on ensuring that the butadiene produced meets the stringent quality requirements necessary for its use in various applications. This specification outlines the critical parameters that must be met to ensure the consistent performance and reliability of the final products.At the heart of the bright light butadiene specification is the need to maintain a high degree of purity. Impurities, even at trace levels, can have a significant impact on the performance and stability of the butadiene-based materials. The specification, therefore, sets strict limits on the allowable levels of various contaminants, such as water, carbon monoxide, and sulfur compounds, to ensure that the butadiene meets the desired standards.Another important aspect of the bright light butadiene specification is the control of the physical properties of the material. This includesparameters such as specific gravity, boiling point, and vapor pressure, all of which can have a direct impact on the handling, transportation, and storage of the butadiene. Ensuring that these properties fall within the specified ranges is crucial to maintaining the safety and efficiency of the supply chain.In addition to the physical properties, the specification also addresses the chemical composition of the butadiene itself. This includes the precise control of the isomeric content, as the ratio of the various butadiene isomers can influence the performance of the final products. The specification, therefore, sets guidelines for the acceptable levels of the cis- and trans-1,3-butadiene isomers, as well as any other minor components that may be present.The implementation of the bright light butadiene specification is not just a matter of ensuring product quality; it also plays a vital role in the sustainability and environmental stewardship of the chemical industry. By setting strict limits on the impurities and contaminants, the specification helps to minimize the environmental impact of the butadiene production and use, reducing the potential for emissions, waste, and other environmental concerns.The development and adoption of the bright light butadiene specification is a collaborative effort involving various stakeholders, including chemical manufacturers, industry associations, andregulatory bodies. This process ensures that the specification remains relevant and responsive to the evolving needs of the market, as well as the changing landscape of environmental and safety regulations.As the demand for butadiene-based materials continues to grow, the importance of the bright light butadiene specification will only become more pronounced. By providing a clear and comprehensive framework for the production and delivery of high-quality butadiene, this specification serves as a critical foundation for the ongoing innovation and development of the chemical industry, ultimately contributing to the advancement of modern society.。
流动化学英语

流动化学英语Fluid Chemistry: An Exploration of the Dynamic Nature of Chemical ProcessesThe realm of chemistry is a captivating and ever-evolving landscape, where the intricate dance of atoms and molecules captivates the minds of scientists and enthusiasts alike. At the heart of this dynamic field lies the concept of fluid chemistry, a fascinating realm that explores the dynamic and ever-changing nature of chemical processes. From the cascading reactions in living organisms to the intricate choreography of industrial processes, fluid chemistry offers a unique perspective on the fundamental principles that govern the transformation of matter.At its core, fluid chemistry is the study of chemical systems that are in a constant state of flux, where the composition, structure, and properties of the reactants and products are in a perpetual state of change. This fluidity, or dynamism, is a hallmark of many chemical processes, and it is this very quality that makes the field of fluid chemistry so intriguing and challenging.One of the primary aspects of fluid chemistry is the study of reactionkinetics, the science that explores the rates and mechanisms of chemical reactions. In a fluid system, these rates can be influenced by a multitude of factors, including temperature, pressure, the presence of catalysts, and the concentration of reactants. By understanding the intricate interplay of these variables, scientists can gain valuable insights into the underlying principles that govern the behavior of chemical systems.Another key facet of fluid chemistry is the exploration of transport phenomena, the study of how materials and energy move within a system. In a fluid environment, the movement of molecules, ions, and even entire phases can have a profound impact on the overall behavior of a chemical system. The study of diffusion, convection, and other transport processes is crucial in understanding the dynamics of chemical reactions, as well as in the design and optimization of industrial processes.The applications of fluid chemistry are far-reaching and diverse, spanning a wide range of industries and disciplines. In the realm of biology, fluid chemistry plays a critical role in the understanding of cellular processes, where the intricate dance of biomolecules is essential for the maintenance and regulation of life. In the field of materials science, fluid chemistry is integral to the development of advanced materials, from self-healing polymers to responsive gels.Moreover, fluid chemistry is at the heart of many industrial processes, from the production of pharmaceuticals and fuels to the purification of water and the treatment of waste. By understanding the dynamic nature of these processes, engineers and scientists can develop more efficient, sustainable, and environmentally-friendly methods of production, ultimately contributing to the betterment of our world.The study of fluid chemistry is not without its challenges, however. The inherent complexity of these dynamic systems can make them difficult to model and predict, requiring the integration of various scientific disciplines, from physics and mathematics to computer science and engineering. Yet, it is precisely this complexity that makes the field of fluid chemistry so captivating and rewarding.As we delve deeper into the world of fluid chemistry, we uncover a tapestry of interconnected phenomena, where the seemingly simple transformations of matter are revealed to be intricate and fascinating. From the cascading reactions in the human body to the intricate processes that drive the global economy, the study of fluid chemistry offers a unique and invaluable perspective on the dynamic nature of our world.In conclusion, the field of fluid chemistry is a testament to the enduring curiosity and ingenuity of the human spirit. By exploring the ever-changing landscapes of chemical processes, we gain adeeper understanding of the fundamental principles that govern the natural world, and we are empowered to harness this knowledge to create a better, more sustainable future for all.。
化学工程与工艺专业英语课后习题参考答案
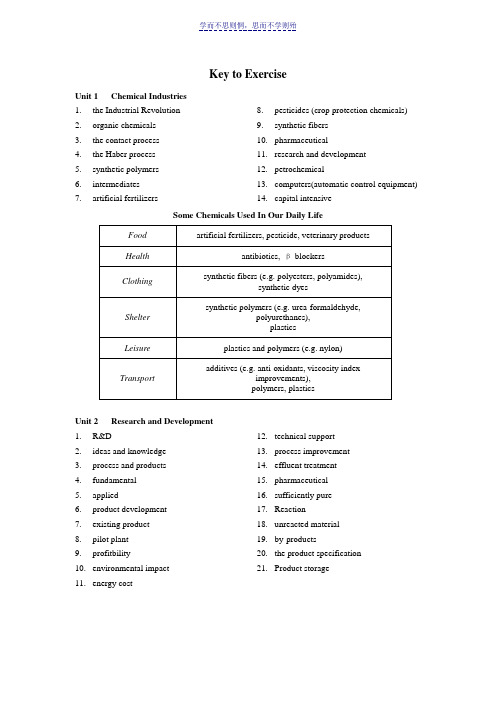
学而不思则惘,思而不学则殆Key to Exercise Unit 1 Chemical Industries1.the Industrial Revolutionanic chemicals3.the contact process4.the Haber process5.synthetic polymers6.intermediates7.artificial fertilizers 8.pesticides (crop protection chemicals)9.synthetic fibers10.pharmaceutical11.research and development12.petrochemicalputers(automatic control equipment)14.capital intensiveSome Chemicals Used In Our Daily LifeUnit 2 Research and Development1.R&D2.ideas and knowledge3.process and products4.fundamental5.applied6.product development7.existing product8.pilot plant9.profitbility10.environmental impact11.energy cost 12.technical support13.process improvement14.effluent treatment15.pharmaceutical16.sufficiently pure17.Reaction18.unreacted material19.by-products20.the product specification21.Product storageUnit 3 Typical Activities of Chemical Engineers1.Mechanical2.electrical3.civil4.scale-upmercial-size6.reactors7.distillation columns8.pumps9.control and instrumentation10.mathematics11.industry12.academia13.steam 14.cooling water15.an economical16.to improve17.P&I Drawings18.Equipment Specification Sheets19.Construction20.capacity and performance21.bottlenecks22.Technical Sales23.new or improved24.engineering methods25.configurationsUnit 4 Sources of Chemicals1.inorganic chemicals2.derive from (originate from)3.petrochemical processes4.Metallic ores5.extraction process6.non-renewable resource7.renewable sources8.energy source9.fermentation process10.selective 11.raw material12.separation and purification13.food industry14.to be wetted15.Key to success16.Crushing and grinding17.Sieving18.Stirring and bubbling19.Surface active agents20.OverflowingUnit 5 Basic Chemicals 1. Ethylene 2. acetic acid 3.4. Polyvinyl acetate5. Emulsion paintUnit 6 Chlor-Alkali and Related Processes 1. Ammonia 2. ammonia absorber 3. NaCl & NH 4OH 4.5. NH 4Cl6. Rotary drier7. Light Na 2CO 3Unit 7 Ammonia, Nitric Acid and Urea 1. kinetically inert 2. some iron compounds 3. exothermic 4. conversion 5. a reasonable speed 6. lower pressures 7. higher temperatures 8.9. energy 10. steam reforming 11. carbon monoxide 12. secondary reformer 13. the shift reaction 14. methane 15. 3:1Unit 8 Petroleum Processing 1. organic chemicals 2. H:C ratios3. high temperature carbonization4. crude tar5. pyrolysis6. poor selectivity7. consumption of hydrogen8. the pilot stage9. surface and underground 10.fluidized bed 11. Biotechnology 12. sulfur speciesUnit 9 PolymersUnit 10 What Is Chemical EngineeringMicroscale (≤10-3m)●Atomic and molecular studies of catalysts●Chemical processing in the manufacture of integrated circuits●Studies of the dynamics of suspensions and microstructured fluidsMesoscale (10-3-102m)●Improving the rate and capacity of separations equipment●Design of injection molding equipment to produce car bumpers madefrom polymers●Designing feedback control systems for bioreactorsMacroscale (>10m)●Operability analysis and control system synthesis for an entire chemicalplant●Mathematical modeling of transport and chemical reactions ofcombustion-generated air pollutants●Manipulating a petroleum reservoir during enhanced oil recoverythrough remote sensing of process data, development and use of dynamicmodels of underground interactions, and selective injection of chemicalsto improve efficiency of recoveryUnit 12 What Do We Mean by Transport Phenomena?1.density2.viscosity3.tube diameter4.Reynolds5.eddiesminar flow7.turbulent flow 8.velocity fluctuations9.solid surface10.ideal fluids11.viscosity12.Prandtl13.fluid dynamicsUnit 13 Unit Operations in Chemical Engineering 1. physical 2. unit operations 3. identical 4. A. D. Little 5. fluid flow6. membrane separation7. crystallization8. filtration9. material balance 10. equilibrium stage model 11. Hydrocyclones 12. Filtration 13. Gravity 14. VaccumUnit 14 Distillation Operations 1. relative volatilities 2. contacting trays 3. reboiler4. an overhead condenser5. reflux6. plates7. packing8.9. rectifying section 10. energy-input requirement 11. overall thermodynamic efficiency 12. tray efficiencies 13. Batch operation 14. composition 15. a rectifying batch 1 < 2 < 3Unit 15 Solvent Extraction, Leaching and Adsorption 1. a liquid solvent 2. solubilities 3. leaching 4. distillation 5. extract 6. raffinate 7. countercurrent 8. a fluid 9. adsorbed phase 10. 400,000 11. original condition 12. total pressure 13. equivalent numbers 14. H + or OH –15. regenerant 16. process flow rates17. deterioration of performance 18. closely similar 19. stationary phase 20. mobile phase21. distribution coefficients 22. selective membranes 23. synthetic24. ambient temperature 25. ultrafiltration26. reverse osmosis (RO).Unit 16 Evaporation, Crystallization and Drying 1. concentrate solutions 2. solids 3. circulation 4. viscosity 5. heat sensitivity 6. heat transfer surfaces 7. the long tube8. multiple-effect evaporators 9.10. condensers 11. supersaturation 12. circulation pump 13. heat exchanger 14. swirl breaker 15. circulating pipe 16. Product17. non-condensable gasUnit 17 Chemical Reaction Engineering1.design2.optimization3.control4.unit operations (UO)5.many disciplines6.kinetics7.thermodynamics,8.fluid mechanics9.microscopic10.chemical reactions 11.more valuable products12.harmless products13.serves the needs14.the chemical reactors15.flowchart16.necessarily17.tail18.each reaction19.temperature and concentrations20.linearUnit 18 Chemical Engineering Modeling1.optimization2.mathematical equations3.time4.experiments5.greater understanding6.empirical approach7.experimental design8.differing process condition9.control systems 10.feeding strategies11.training and education12.definition of problem13.mathematical model14.numerical methods15.tabulated or graphical16.experimental datarmation1.the preliminary economics2.technological changes3.pilot-plant data4.process alternatives5.trade-offs6.Off-design7.Feedstocks 8.optimize9.plant operations10.energy11.bottlenecking12.yield and throughput13.Revamping14.new catalystUnit 19 Introduction to Process Design1. a flowsheet2.control scheme3.process manuals4.profit5.sustainable industrial activities6.waste7.health8.safety9. a reactor10.tradeoffs11.optimizations12.hierarchyUnit 20 Materials Science and Chemical Engineering1.the producing species2.nutrient medium3.fermentation step4.biomass5.biomass separation6.drying agent7.product8.water9.biological purificationUnit 21 Chemical Industry and Environment1.Atmospheric chemistry2.stratospheric ozone depletion3.acid rain4.environmentally friendly products5.biodegradable6.harmful by-product7.efficiently8.power plant emissions 9.different plastics10.recycled or disposed11.acidic waste solutionsanic components13.membrane technology14.biotechnology15.microorganisms。
高分子材料英文
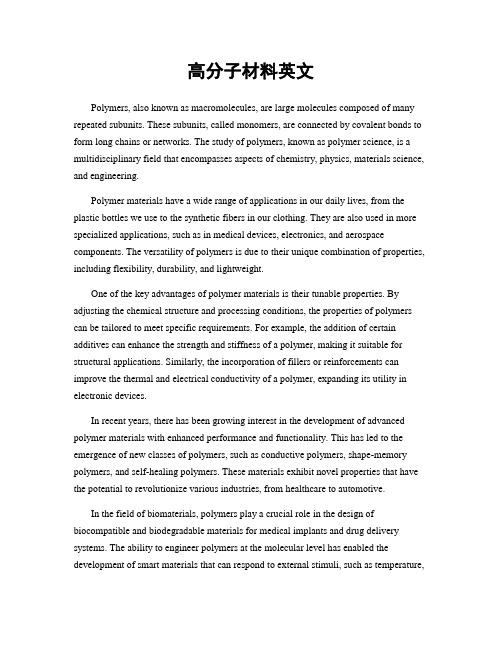
高分子材料英文Polymers, also known as macromolecules, are large molecules composed of many repeated subunits. These subunits, called monomers, are connected by covalent bonds to form long chains or networks. The study of polymers, known as polymer science, is a multidisciplinary field that encompasses aspects of chemistry, physics, materials science, and engineering.Polymer materials have a wide range of applications in our daily lives, from the plastic bottles we use to the synthetic fibers in our clothing. They are also used in more specialized applications, such as in medical devices, electronics, and aerospace components. The versatility of polymers is due to their unique combination of properties, including flexibility, durability, and lightweight.One of the key advantages of polymer materials is their tunable properties. By adjusting the chemical structure and processing conditions, the properties of polymers can be tailored to meet specific requirements. For example, the addition of certain additives can enhance the strength and stiffness of a polymer, making it suitable for structural applications. Similarly, the incorporation of fillers or reinforcements can improve the thermal and electrical conductivity of a polymer, expanding its utility in electronic devices.In recent years, there has been growing interest in the development of advanced polymer materials with enhanced performance and functionality. This has led to the emergence of new classes of polymers, such as conductive polymers, shape-memory polymers, and self-healing polymers. These materials exhibit novel properties that have the potential to revolutionize various industries, from healthcare to automotive.In the field of biomaterials, polymers play a crucial role in the design of biocompatible and biodegradable materials for medical implants and drug delivery systems. The ability to engineer polymers at the molecular level has enabled the development of smart materials that can respond to external stimuli, such as temperature,pH, or light. These stimuli-responsive polymers have applications in controlled drug release, tissue engineering, and diagnostics.Furthermore, the use of polymers in additive manufacturing, also known as 3D printing, has opened up new possibilities for the rapid prototyping and production of complex geometries. This technology allows for the fabrication of custom-designed parts with precise control over material properties, making it particularly attractive for the aerospace and automotive industries.In conclusion, high-performance polymer materials have revolutionized the way we design and manufacture products across various sectors. Their tunable properties, versatility, and ability to be tailored for specific applications make them indispensable in modern technology and industry. As research in polymer science continues to advance, we can expect to see even more innovative and sustainable polymer materials in the future.。
纳米多功能衣服作文英语
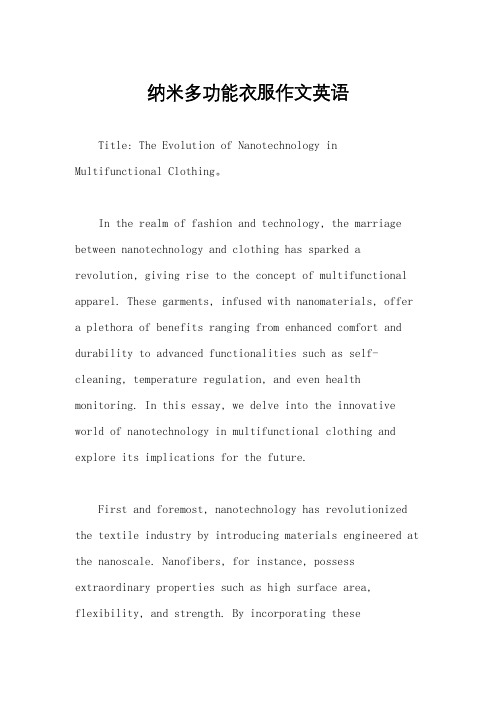
纳米多功能衣服作文英语Title: The Evolution of Nanotechnology in Multifunctional Clothing。
In the realm of fashion and technology, the marriage between nanotechnology and clothing has sparked a revolution, giving rise to the concept of multifunctional apparel. These garments, infused with nanomaterials, offer a plethora of benefits ranging from enhanced comfort and durability to advanced functionalities such as self-cleaning, temperature regulation, and even health monitoring. In this essay, we delve into the innovative world of nanotechnology in multifunctional clothing and explore its implications for the future.First and foremost, nanotechnology has revolutionized the textile industry by introducing materials engineered at the nanoscale. Nanofibers, for instance, possess extraordinary properties such as high surface area, flexibility, and strength. By incorporating thesenanofibers into clothing, manufacturers can create fabrics that are not only lightweight and breathable but alsowater-repellent and quick-drying. This is particularly advantageous in outdoor apparel, where protection against the elements is crucial.Moreover, the integration of nanomaterials has endowed clothing with self-cleaning capabilities. Nano-coatings applied to fabric surfaces repel dirt, oil, and water, thereby preventing stains and odors from adhering. This not only reduces the need for frequent washing but also prolongs the lifespan of the garment. Imagine a world where spills and splashes simply roll off your clothes, leaving them pristine and fresh—a reality made possible by nanotechnology.Furthermore, nanotechnology has paved the way for smart textiles capable of sensing and responding to external stimuli. By embedding sensors and conductive materials at the nanoscale, clothing can monitor vital signs, detect environmental changes, and even deliver therapeutic treatments. For instance, wearable biosensors integratedinto athletic apparel can track athletes' performance metrics such as heart rate, respiration, and hydration levels in real-time, optimizing training routines and preventing injuries.Additionally, nanotechnology enables the creation of thermoregulating fabrics that adapt to the wearer's body temperature. Phase change materials (PCMs) embedded within the fabric absorb, store, and release heat as needed, ensuring optimal comfort in varying climates. This not only enhances wearer satisfaction but also reduces the reliance on traditional heating and cooling systems, thus promoting energy efficiency and sustainability.Furthermore, the advent of nanotechnology has usheredin a new era of personalized clothing tailored toindividual preferences and needs. By leveraging nanomaterials with tunable properties, such as shape memory alloys and responsive polymers, garments can dynamically adjust their fit, style, and functionality. This customization extends beyond aesthetics to encompass functional enhancements, enabling users to tailor theirclothing for specific activities or environments.In conclusion, the integration of nanotechnology into multifunctional clothing represents a paradigm shift in the fashion industry, offering unparalleled comfort, functionality, and sustainability. From self-cleaning fabrics to smart textiles capable of monitoring health metrics, the possibilities are endless. As nanotechnology continues to advance, we can expect to see further innovations that blur the boundaries between fashion and technology, enhancing our lives in ways we never thought possible.。
关于生物的英语作文
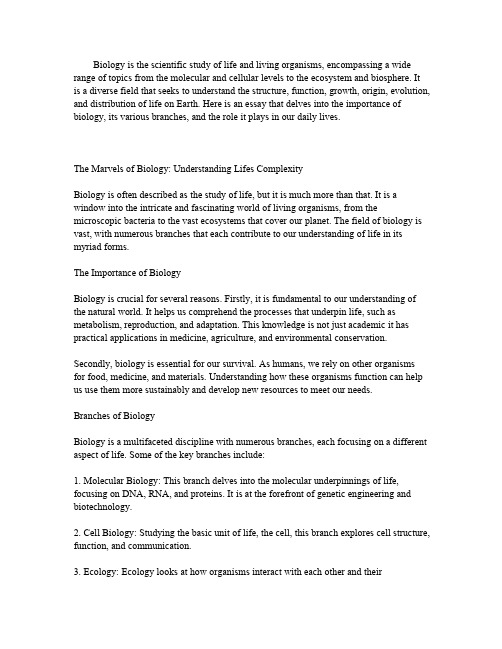
Biology is the scientific study of life and living organisms,encompassing a wide range of topics from the molecular and cellular levels to the ecosystem and biosphere.It is a diverse field that seeks to understand the structure,function,growth,origin,evolution, and distribution of life on Earth.Here is an essay that delves into the importance of biology,its various branches,and the role it plays in our daily lives.The Marvels of Biology:Understanding Lifes ComplexityBiology is often described as the study of life,but it is much more than that.It is a window into the intricate and fascinating world of living organisms,from the microscopic bacteria to the vast ecosystems that cover our planet.The field of biology is vast,with numerous branches that each contribute to our understanding of life in its myriad forms.The Importance of BiologyBiology is crucial for several reasons.Firstly,it is fundamental to our understanding of the natural world.It helps us comprehend the processes that underpin life,such as metabolism,reproduction,and adaptation.This knowledge is not just academic it has practical applications in medicine,agriculture,and environmental conservation. Secondly,biology is essential for our survival.As humans,we rely on other organisms for food,medicine,and materials.Understanding how these organisms function can help us use them more sustainably and develop new resources to meet our needs. Branches of BiologyBiology is a multifaceted discipline with numerous branches,each focusing on a different aspect of life.Some of the key branches include:1.Molecular Biology:This branch delves into the molecular underpinnings of life, focusing on DNA,RNA,and proteins.It is at the forefront of genetic engineering and biotechnology.2.Cell Biology:Studying the basic unit of life,the cell,this branch explores cell structure, function,and communication.3.Ecology:Ecology looks at how organisms interact with each other and theirenvironment.It is vital for understanding and managing ecosystems.4.Evolutionary Biology:This branch traces the history of life on Earth,examining how species have evolved over time.5.Physiology:Focusing on how organisms function,physiology looks at the processes that occur within living organisms to sustain life.6.Genetics:Genetics is the study of heredity and the variation of inherited characteristics. It is a cornerstone of modern medicine and agriculture.Biology in Daily LifeBiology is not just confined to laboratories and academic institutions.It is a part of our daily lives.From the food we eat,which is the result of agricultural biology,to the medicines we take,which are often derived from biological research,biology impacts us in countless ways.Moreover,understanding biology can help us address some of the most pressing issues facing our world today,such as climate change,disease outbreaks,and biodiversity loss. By studying biology,we can develop strategies to mitigate these challenges and ensure a sustainable future.ConclusionIn conclusion,biology is a field of endless wonder and discovery.It is the key to unlocking the mysteries of life and is essential for our understanding of the world around us.As we continue to explore the depths of biological knowledge,we open doors to new possibilities and solutions that can shape our future for the better.Whether its through the development of new medicines,the conservation of endangered species,or the advancement of sustainable technologies,the study of biology is a journey into the very essence of what it means to be alive.。
CO2-responsive polymer
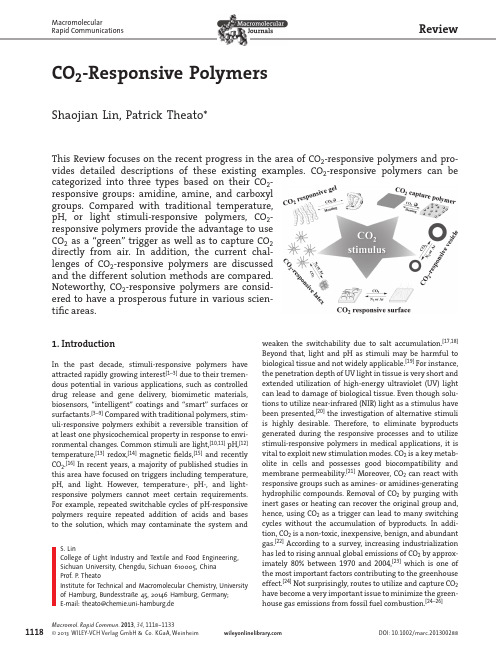
MacromolecularRapid CommunicationsReview1118D OI : 10.1002/marc .201300288 1.IntroductionI n the past decade, stimuli-responsive polymers haveattracted rapidly growing interest[ 1–3 ] due to their tremen-dous potential in various applications, such as controlled drug release and gene delivery, biomimetic materials, biosensors, “intelligent’’ coatings and “smart’’ surfaces orsurfactants. [ 3–9 ] Compared with traditional polymers, stim-uli-responsive polymers exhibit a reversible transition ofat least one physicochemical property in response to envi-ronmental changes. Common stimuli are light, [ 10 , 11 ]pH, [ 12 ]temperature, [ 13 ]redox, [ 14 ]magnetic fi elds, [ 15 ] and recentlyCO 2.[ 16 ] In recent years, a majority of published studies in this area have focused on triggers including temperature, pH, and light. However, temperature-, pH-, and light-responsive polymers cannot meet certain requirements. For example, repeated switchable cycles of pH-responsive polymers require repeated addition of acids and bases to the solution, which may contaminate the system andweaken the switchability due to salt accumulation. [ 17 , 18 ]Beyond that, light and pH as stimuli may be harmful tobiological tissue and not widely applicable. [ 19 ] For instance,the penetration depth of UV light in tissue is very short and extended utilization of high-energy ultraviolet (UV) light can lead to damage of biological tissue. Even though solu-tions to utilize near-infrared (NIR) light as a stimulus havebeen presented, [ 20 ] the investigation of alternative stimuliis highly desirable. Therefore, to eliminate byproducts generated during the responsive processes and to utilize stimuli-responsive polymers in medical applications, it is vital to exploit new stimulation modes. CO 2 is a key metab-olite in cells and possesses good biocompatibility andmembrane permeability. [ 21 ] Moreover, CO 2 can react withresponsive groups such as amines- or amidines-generating hydrophilic compounds. Removal of CO 2 by purging with inert gases or heating can recover the original group and, hence, using CO 2 as a trigger can lead to many switching cycles without the accumulation of byproducts. In addi-tion, CO 2 is a non-toxic, inexpensive, benign, and abundantgas. [ 22 ] According to a survey, increasing industrialization has led to rising annual global emissions of CO 2 by approx-imately 80% between 1970 and 2004, [ 23 ] which is one of the most important factors contributing to the greenhouseeffect. [ 24 ] Not surprisingly, routes to utilize and capture CO 2 have become a very important issue to minimize the green-house gas emissions from fossil fuel combustion. [ 24–26 ]T his Review focuses on the recent progress in the area of CO 2-responsive polymers and pro-vides detailed descriptions of these existing examples. CO 2-responsive polymers can be categorized into three types based on their CO 2 -responsive groups: amidine, amine, and carboxyl groups. Compared with traditional temperature,pH, or light stimuli-responsive polymers, CO 2 -responsive polymers provide the advantage to use CO 2 as a “green” trigger as well as to capture CO 2 directly from air. In addition, the current chal-lenges of CO 2-responsive polymers are discussed and the different solution methods are compared.Noteworthy, CO 2-responsive polymers are consid-ered to have a prosperous future in various scien-tifi c areas.C O 2-Responsive PolymersShaojian Lin ,Patrick Theato *S.LinCollege of Light Industry and Textile and Food Engineering, Sichuan University, Chengdu, Sichuan 610005, China P rof. P . TheatoInstitute for Technical and Macromolecular Chemistry, University of Hamburg, Bundesstraße 45, 20146 Hamburg, Germany; E-mail: theato@chemie.uni-hamburg.deMacromol. Rapid Commun. 2013, 34, 1118−1133© 2013 WILEY-VCH Verlag GmbH & Co. KGaA, WeinheimMacromolecularRapid CommunicationsCO 2-Responsive Polymerswww.mrc-journal.de1119activities on CO 2-responsive polymers gained momentum just within recent years.C urrently, responsive mechanisms of CO 2-responsive polymers can be divided into three categories according totheir responsive behavior. The fi rst group features respon-sive groups such as amidine or amine in CO 2-responsive polymers that possess a high nucleophilicity or basicity. Therefore, their basic moieties can form amidinium bicar-bonates, [ 54 ] zwitterionic adducts, [ 55 ] or ammonium carba-mates [ 56 ] upon successful capture of CO 2. In the second category, the responsive groups (amidine, amine, or car-boxyl) switch from a neutral moiety to a charged species upon the addition of CO 2 due to protonation of respon-sive groups. [ 8 , 21 , 57 , 58 ] Last but not least, the third category consists of responsive groups, i.e., amines, that can react with CO 2 to form an “ammonium carbamate salt bridge,”which results in a cross-link. [ 59 , 60 ]I n this review, we focus on CO 2-responsive polymers that are currently available. These polymers can be cat-egorized into three types based on their functional group (amidine, amine, or carboxyl group) and par-ticular emphasis is laid on the latest developments in CO 2-responsive polymers to emphasize the molecular design of such polymers. Moreover, we forecast that CO 2 -responsive polymers will be widely utilized in a variety of advanced materials science applications.Consequently,CO 2 -responsive polymers and CO 2cap-ture materials have been extensively investigated. Since the last century, traditional aqueous amine solutions have been used to absorb CO 2, however, technologi-cally, this method requires complex processing, is costly,and involves large facilities. [ 27 , 28 ] This has encouragedresearchers to exploit new CO 2 capture materials. [ 29 ]Inthe past decades, various new CO 2 capture materials were devised and applied in industries, such as the carbo-naceous adsorbents, [ 30 , 31 ] porous structure materials, [ 32 ] metal oxides, [ 30 , 33 ] metal–organic frameworks, [ 34 ]organicsolids, [ 35 ] ionic liquids and poly(ionic liquid)s, [ 36–40 ]and CO 2-responsive solvents.[ 41 ] Especially, CO 2-responsivesolvents attracted enormous attentions owing to their unique switchable and versatile properties. Jessop and co-workers investigated CO 2-responsive solvents for the fi rst time and demonstrated that some CO 2-respon-sive solvents can be switched between a non-polar form and a polar form by the stimulation of CO 2,[ 41,42 ] followed by others. [ 43 − 46 ] Accordingly, some CO 2-respon-sive solvents can switch reversibly back and forthbetween a hydrophilic and hydrophobic state by intro-ducing and releasing CO 2gas.[ 47–51 ] Subsequently, Helde-brant and co-workers [ 41 ] developed the fi rst example ofCO 2 -responsive solvents that could capture CO 2directly from air. Recently, Wang and Baker’s groups published a series of CO 2 -responsive solvents for CO 2monitoring,as a facile CO 2detection approach. [ 52 , 53 ] Inspired by these attractive characteristics of CO 2-responsive sol-vents, research activities on CO 2-responsive polymers have taken momentum recently. Investigations have focused on introducing the switchable groups used in CO 2-responsive solvents onto polymer chains to pre-pare the CO 2 -responsive polymers. Similar to other stimuli-responsive polymers, CO 2-responsive polymers also show a reversible switching of at least a physico-chemical property when adding and removing CO 2,i.e.,when applying the stimulus. However, in contrast to other pH changes, the responsive process occurring when CO 2 is added does not accumulate byproducts. Mean-while, CO 2 is benign biocompatible and hence, compared with temperature or light stimuli-responsive polymers, CO 2-responsive polymers are better candidates for appli-cation in drug and gene delivery or biomimetic mate-rials. In addition, in contrast to other stimuli-responsive polymers, CO 2-responsive polymers can be used in other important areas, for example, to CO 2 capture. Using polymers to capture CO 2 from air is one of the simplest, economically effective methods for CO 2 recovery and utilization. Therefore, CO 2-responsive polymers received enormous attention, owing to CO 2 as a “green” trigger.Actually, already in 2004, Endo et al. [ 54 ]publishedthe fi rst example of a CO 2-responsive polymer based on amidine units to capture CO 2. However, researchP atrick Theato studied chemistry at the University of Mainz and the University of Massachusetts, Amherst, and obtained his Ph.D. degree from the University of Mainzin 2001. After postdoctoral studies at Seoul National University supported by Feodor Lynen Postdoctoral Research Fellowship and at Stanford University, he completed his Habilitation at the University of Mainz in 2007. From 2009 to 2012, he held a joint appointment at Seoul National University within the WCU program. In 2011, he accepted a prize senior lec-tureship at the University of Sheffi eld. Shortly after he moved to University of Hamburg as an associate professor for polymer chemistry.S haojian Lin received his B. E. in Textile Engineering under the supervision of Prof. Bojun Xi from the Shaoxing University (China) in 2009, working on fi ber materials. He then went to Sichuan University (China) for his Master’s thesis to work in the group of Prof. Jianwu Lan on poly(ester-ether) elas-tomer fi bers with excellent thermal stability. Subsequently, he joined the group of Prof. Patrick Theato at University of Hamburg (Germany) for his Ph.D. in 2013 with a focus on CO 2-responsive polymers and fi bers. Macromol. Rapid Commun. 2013, 34, 1118−1133© 2013 WILEY-VCH Verlag GmbH & Co. KGaA, WeinheimS. Lin and P . TheatoMacromolecularRapid Communicationswww.mrc-journal.de1120corresponding low-molecular-weight amidine structures at ambient temperature and could be applied as materials for reversible fi xation–release of CO 2. A s an alternative, Zhou and co-workers [ 55 ]synthesizeda novel type of polymer poly(NHC) based on N -heterocy-clic carbene (NHC) moieties for a CO 2 capture (Figure 1 c ). Compared with Endo’s polymers, their polymer can cap-ture equal amounts of CO 2 in a much shorter time. This higher CO 2 capturing efficiency and faster capture rate can be ascribed to the ability of NHCs to adsorb CO 2at relatively low CO 2 concentration. Moreover, the formed carboxylate, poly(NHC-CO 2), shows a better thermal sta-bility. Thermogravimetric analysis (TGA) reveals that the polymer poly(NHC-CO 2) begin losing weight above 120 °C and a total weight lost of 6.22 wt% is observed between 120 ° C and 160 ° C . Therefore, poly(NHC) is an ideal CO 2 absorbent candidate in the conventional industrial pro-cess for recovering CO 2gas. 2.1.2.CO 2-Responsive GelsNagai and co-workers [ 62] prepared a gel consisting of poly(allylamine) (PAA) with DBU side groups and CO 2as gellant in 2011. The gel formed by a cross-linking reaction between the PAA-DBU polymer with CO 2. This kind of gel possesses very good properties to absorb CO 2 and it can reversibly absorb CO 2 at 25 ° C and release the absorbed CO 2 in N 2 atmosphere at 120° C . The repeatedly absorb–release processes do not damage the gel properties. The2. Overview of CO 2-ResponsivePolymers2.1.CO 2 -Responsive Polymers Based on Amidine Groups T he use of amidine is a type of CO 2-switchable mole-cule was reported by Jessop and co-workers [ 16 ] and Endoet al. [ 54 ] Endo et al. [ 54 ] fi rst published a polymer containing amidine groups that was able to directly capture CO 2from air, whereas the group of Jessop reported a polarity switch-able solvent based on an amidine group. They devised a system, in which a nonpolar solvent molecule is converted to a polar solvent molecule upon exposure to an atmos-phere of CO 2. The original nonpolar state could be recov-ered when bubbling an inert gas like N 2 or Ar through thesolution to expel CO 2.[ 16 ] These studies have been the inspi-ration for other researchers to investigate CO 2-responsivepolymers on the basis of amidine groups. In the following, we categorize the polymers into fi ve types, based on their structures or their applications. 2.1.1.CO 2 -Responsive Polymers for CO 2CaptureT he development of polymers for an efficient capturing of CO 2 from industrial waste gases is extremely mean-ingful in respect to the utilization of CO 2 and decrease the greenhouse effect. Hence, various responsive polymersfor CO 2 fixation have been developed. Endo et al. [ 54 ] fi rst reported a new type of polymers on the basis of amidine moieties that can reversibly capture CO 2 in solution and in solid state. In this paper, N -methyltetrahydropyrimidine (MTHP), containing an amidine structure, was attached to a polymer. They synthesized a polystyrenic derivative bearing an amidine moiety, poly(THPSt) in Figure 1 a . It turned out that this type polymer has a higher CO 2 fi xation effi ciency and can release CO 2 at lower temperature com-pared with ammonium carbamates derived from primary or secondary amines. In order to further improve the CO 2 capture ability, the effect of the functional comonomer N -vinylacetamide (NVA) on CO 2 fi xation was examined. Notably, the copolymer poly(THPSt 50-c o -NVA 50)exhibited a higher CO 2 fixation ability than poly(THPSt). This can be attributed to the higher CO 2 permeability of poly(NVA). Therefore, this kind of polymer might be a suitable mate-rial for CO 2 storage to remove CO 2 from industrial waste gases. However, the synthesis of the polymers bearing the MTHP moiety is a cumbersome process. Consequently,Endo and co-workers [ 61 ] developed a new type of poly-mers for capturing CO 2, which is based on inexpensive and accessible amidines (Figure 1 b ). They synthesized copolymers bearing (1,8-diazabicyclo[5.4.0]undec-7-ene) (DBU) and (1,5-diazabicyclo[4.3.0]-non-5-ene) (DBN) moie-ties to fi x CO 2 from air. These copolymers can hold trapped CO 2 at 25 ° C and release CO 2 at 120 ° C via a N 2 flow. The results showed that these cyclic amidine-functionalized copolymers exhibit a better ability to capture CO 2 than theF igure 1. T he molecular structures of different CO 2-capturing/CO 2-responsive polymers: redrawn from the literature: a) [ 54 ];b)[ 61 ];c) [ 55 ]; and d) [ 8 ].Macromol. Rapid Commun. 2013, 34, 1118−1133© 2013 WILEY-VCH Verlag GmbH & Co. KGaA, WeinheimMacromolecularRapid CommunicationsCO 2-Responsive Polymerswww.mrc-journal.de 11212.1.3.CO 2-Responsive HomopolymersIn 2011, Feng and co-workers [ 8 ]published a paper on a novel smart homopolymer that is responsive to CO 2.To avoid a cumbersome synthesis, Feng and co-workers used a facile way with just three simple steps to prepare an amidine-based polymer with suitable hydrophobicity by reversible addition–fragmentation chain transfer (RAFT) polymerization and “click” reaction (Figure 1 d ). While the homopolymer is not soluble in pure water, it could be dis-solved in common organic solvents, forming light yellow solutions, which implies that this polymer has a hydro-phobic character. When the homopolymer is put into pure CHCl 3 /water (1:1, v/v) mixture in the absence and presence of CO 2, it can observe that polymer undergoes a hydrophobic–hydrophilic transition upon loading with CO 2 (Figure 2 ). In the absence of CO 2, the polymer solution separates into two phases, with the lower organic layer containing the hydrophobic yellowish polymer. When bub-bling CO 2 into the polymer solution, the upper aqueous layer turns light yellow while the lower phase becomescolorless, indicating that the polymer changes from hydrophobic to hydrophilic and therefore dissolves in the water layer. This phenomenon can be explained by the fact that the amidine-based polymer changes from a neutral and hydrophobic to a charged and hydrophilic polyelectro-lyte after bubbling with CO 2. 2.1.4.CO 2-Responsive Copolymersand Block CopolymersF eng and co-workers [ 57 ]synthesizeda pyrene-modifi ed CO 2-responsive copolymer (p-AP) to improve disper-sion of single-walled carbon nanotubes (SWNTs). As shown in Figure 3 a , two copolymers p-AP 23 and p-AP 12with varying amounts of amidine groups were synthesized. The investigation of dispersibility of SWNTs showed that p-AP 12 results in stable dispersions in both dichloromethane (DCM) and N ,N -dimethylformamide (DMF) due to its moderate polarity and physical inter-actions. Next, similar to their previous work, the authors examined the hydro-phobic–hydrophilic transition and dis-persion state of p-AP 12/SWNT hybrids in a DCM/water (1:1, v/v) solution by the stimulation of CO 2 . Before CO 2addi-tion, only a fl uorescent signal of the emission of pyrene in the DCM phase is observed, while no fl uorescent signalsmaximum absorption CO 2 ability of this kind of gel is four times larger than that of PAA aqueous solution and 2.8 times larger than that of most commonly used absor-bents at 25 ° C . Therefore, this kind of gel might have a bright prospect as a CO 2 absorption material.Figure 2.A)Pure mixed CHCl 3 /water solvent; B) before purgingwith CO 2 ; and C) after purging with CO 2. Reproduced with permis-sion. [ 8 ]Copyright 2011, Royal Society of Chemistry.Figure 3. a ) The synthesis processes of p-AP and b,c) fl uorescence emission spectrum of p-AP 12 /SWNT hybrids before (b)/after (c) passing CO 2 . Reproduced with permission. [ 57 ]Copyright 2013, John Wiley and Sons.Macromol. Rapid Commun. 2013, 34, 1118−1133© 2013 WILEY-VCH Verlag GmbH & Co. KGaA, WeinheimS. Lin and P . TheatoMacromolecularRapid Communicationswww.mrc-journal.de1122sharp and instantaneous change in con-ductivity (Figure 4b).I n 2011, Yan et al. [ 21 ] reported a CO 2-responsive block copolymer that formedso-called respirable vesicles becausetheir size could be altered upon CO 2 stimulation. In order to overcome diffi -culties from synthesizing polyamidinedirectly, Yan and co-workers synthe-sized a difunctional monomer, ( N -ami-dino)dodecyl acrylamide (AD), which consists of a highly reactive acrylic group and a pendant amidine group. Using atom transfer radical polymeriza-tion (ATRP) and a poly(ethylene oxide) (PEO) initiator, they prepared the target diblock copolymer PEO- b -poly(AD)at room temperature. Due to its amphiph-ilic character, this block copolymer self-assembles in aqueous solutions into vesicles. The remarkable fi nding was that these vesicles can expand in sizewhen CO 2 is passed through the solu-tion (Figure 5 ). The reason why the vesi-cles still remained intact can be found in the fact that only 37% of amidine sidegroups are protonated. It is important to stress that these vesicles can return to their initial size upon bubbling Ar through the solution, i.e., removal of CO 2 . This reversible are observed in the upper aqueous solution, indicating thatno p-AP 12 /SWNT hybrids are dispersed in the water phase. Upon passing CO 2through the solution, the fl uorescence emission of the two phases is reversed, that is the upper aqueous solution exhibits a strong fl uorescence signal, while the lower DCM phase exhibits only a weak emission caused by the remanent p-AP 12 (Figure3 ). These results clearly showed that p-AP 12 experiences also a hydro-phobic–hydrophilic transition by CO 2 treatment. p-AP 12 /SWNT hybrids could also be well dispersed in water under ultrasonic treatment with CO 2 bubbling. In contrast, dis-persed p-AP 12 /SWNT precipitated from aqueous solution when CO 2 is removed by bubbling N 2 at 60°C . I n 2009, Zhou et al. [ 63] synthesized a novel poly m er by using the Staudinger ligation to attach 4-{5 ′-[1′ ′-(dimethylamino)-ethylideneamino]pentyl}-1-methyl-2-(diphenylphosphino)terephthalate onto poly( p -azidomethylstyrene-co -styrene)(poly(AMS-co -S)(Figure 4 a ). Again, due to the amidine moiety, it repre-sents a polymer that can be switched between a neu-tral and a charged state in solution by bubbling CO 2or N 2 through the solution. In this paper, the authors used poly(“amidine”MS- c o -S) to study the dynamics of poly-electrolyte solutions. The polymer could be switchedreversibly between a neutral and a charged polycationic state via the alternating introduction of CO 2 and N 2,respectively. This reversible change was confirmed by aFigure 4. R eversible change of a) the molecular structure and b) the solution conduc-tivity of poly(“amidine”MS- c o -S) when purging with CO 2 and N 2 , respectively. Repro-duced with permission. [ 63 ] Copyright 2009, American Chemical Society.Figure 5.T EM images about vesicle sizes in the a) absence or c) presence of CO 2 and DLS data for self-assembled PEO- b -poly(AD)aggregates in the b) absence or d)presence of CO 2.Reproducedwith permission. [ 21 ]Copyright 2011, John Wiley and Sons.Macromol. Rapid Commun. 2013, 34, 1118−1133© 2013 WILEY-VCH Verlag GmbH & Co. KGaA, WeinheimMacromolecularRapid CommunicationsCO 2-Responsive Polymerswww.mrc-journal.de 1123conducted. In this respect, CO 2-responsive polymer latexes have attracted particular attention due to two advantages. First, avoiding the accumulation of electrolytes by addition of acids for anionically stabilized latexes or alkali for cati-onically charged latexes. Utilizing CO 2 as a trigger can pre-vent the production of salt byproducts during a repeatable coagulable/redispersible process. Second, CO 2-responsive polymer latexes are more suitable to be applied in biomed-ical and pharmaceutical applications. In the past years, Jes-sop’s and Zhu’s groups reported on CO 2-responsive polymer latexes based on amidine groups.A s mentioned above, Jessop’s group investigated ami-dine switchable surfactants and logically, they prepared polymer latexes using these switchable surfactants. In 2011, Jessop and co-workers described the preparation of polystyrene (PS) and poly(methyl methacrylate) (PMMA) latexes by emulsion polymerization using cationic ami-dine-based switchable surfactants [ 58 ] ; however, the desta-bilization times of these polymer latexes upon CO 2expo-sure require several hours, which is too slow for practicalpurposes. Shortly after, an improvement was reported on the repeatable aggregation–redispersion of the CO 2-responsive PS latexes. [ 67 ] Neither heating, nor additionof acids or bases is required, only simple bubbling of CO 2 or N 2 is sufficient. The group also found that the redis-persion of polymer latexes is most effective when both the surfactant and the initiator used in the emulsionprocess of expansion and contraction of vesicles inresponse to CO 2 or Ar is called “breathing” vesicles. Basedon this “breathing” characteristic, Yuan and co-workers [ 64 ]further investigated the membrane permeability of vesi-cles. The membrane permeability of vesicles was tested for two kinds of dye-labeled hyperbranched poly(ethylene imine) nanoparticles (PEI-5 and PEI-25 with different sizes) through the membrane after bubbling CO 2.Before addition of CO 2, only the smaller PEI-5 nanoparticles pass through the membrane because the size of the pores in the vesicle is much smaller than that of nanoparticles. However, after stimulation with CO 2 for 10 min, the release rate of the smaller PEI-5 nanoparticles increases signifi cantly because of the enlarged membrane pores in vesicles. Oppositely, the larger PEI-25 still could not yetpass the membrane pores of vesicles. However, furtheraddition of CO 2 to solution results in larger vesicles with much bigger pores and, consequently, PEI-25 nanoparti-cles could pass through the membrane of vesicles. There-fore, this kind of CO 2-responsive vesicle has the potential to be used as selective nanoseparators to sieve complex mixtures of proteins based on their size and hence is par-ticularly interesting for drug or gene delivery.Q uek et al. [ 65] published another study on CO 2-respon-sive block copolymers in 2013. The authors investigated poly(pentafl uorophenyl acrylate) poly(PFPA) homopoly-mers in a post-polymerization modifi cation with com-mercially available amidine and guanidine species, i.e., histamine (HIS) dihydrochloride and L -argininemethyl ester (ARG) dihydrochloride, resulting in thecorresponding amidine and guanidine-based acryla-mido homopolymers (Figure 6 a ). This strategy was then expanded to the synthesis of block copolymers. By RAFT polymerization, either a N ,N -dimethylacrylamide (DMA)or poly(ethylene glycol) methyl ether acrylate (PEGA) was incorporated as a second block (Figure 6 b ). Subsequently, the poly(PFPA) block was reacted with HIS dihydrochlo-ride or ARG dihydrochloride to obtain the functionalized block polymers. It was found that only HIS-based block copolymers exhibit a tunable solubility upon CO 2expo-sure. Therefore, HIS-based block copolymers are able to undergo a reversible assembly–disassembly into spher-ical polymeric micelles by removal or introduction of CO 2, respectively. In contrast to Yan’s work, the HIS-based block has a less hydrophobic character and hence can be transformed into a completely water-soluble block upon exposure with CO 2, thereby resulting in a complete disas-sembly of the micelles into unimers. 2.1.5.CO 2 -Responsive Polymer LatexesThe reversible stabilization of latexes is of industrial importance. [ 66 ] Hence, investigations on polymer latexeswith easily coagulable/redispersible properties have beenFigure 6. T he synthesis processes of a) amidine- and guanidine-based acrylamido homopolymers and b) precursor block poly-mers. Reproduced with permission. [ 65 ] Copyright 2013, John Wiley and Sons.Macromol. Rapid Commun. 2013, 34, 1118−1133© 2013 WILEY-VCH Verlag GmbH & Co. KGaA, WeinheimS. Lin and P . TheatoMacromolecularRapid Communicationswww.mrc-journal.de1124polymerization are based on CO 2 switchable amidine moieties. In order to obtain faster destabilization times of polymer latexes and with a much lower zeta poten-tial, Jessop and co-workers [ 68 ] studied also less basicswitchable surfactants in the emulsion polymerization of PMMA latexes under CO 2 atmosphere. For this, three dif-ferent CO 2-triggered switchable surfactants were inves-tigated and it turned out that aryl amidine and tertiary amine switchable surfactants are more suitable as CO 2 -responsive surfactants for PMMA polymer latexes. As shown in Figure 7 , PMMA latexes will aggregate due to protonation of the CO 2 -responsive group, however, upon removal of CO 2, the surfactants become uncharged and the aggregated latexes are destabilized. It was also found that reducing the initiator concentration can reduce the polymerization rate and that increasing the CO 2pres-sure to 5 atm afforded smaller particles, which provides an easier destabilization of polymer latexes. However, it has to be noted that surfactants based on amidine groups are usually more expensive in comparison with ter-tiary amine surfactants. To address this problem, Jessopet al. [ 69 ] prepared CO 2-responsive PS latexes by utilizing commercially available 2,2 ′-azobis[2-(2-imidazolin-2-yl)propane] (VA-061) as a CO 2-responsive switchable ini-tiator in a surfactant-free emulsion polymerization. The obtained PS latexes are very stable and effective in their reversible aggregation behavior because the amidine group is covalently bound to the particle surface instead of being only physically adsorbed. Accordingly, this CO 2 -responsive PS latex can be aggregated or redispersed by using only Ar or CO 2 gas because of the transition of a hydrophobic into a hydrophilic PS latex surface (Figure 8 ).Z hu et al. [ 70 ]also reported a series of CO 2-responsive polymer latexes on the basis of amidine groups. However, they focused on utilizing a CO 2-responsive monomer,2-methyl-1-(4-vinylbenzyl)-1,4,5,6-tetrahydropyrimidine, which was incorporated into PS chains through a soap-free emulsion copolymerization yielding CO 2-responsivereversible coagulation/redispersion PS latexes (Figure 9 a ).Unfortunately, this polymer latex cannot be assembledfrom dispersion directly. Fortunately, the authors foundthat the latex particles readily coagulated/precipitated after the addition of caustic soda to adjust the pH value and could be redispersed upon exposure to CO 2and ultrasound. Noteworthy, the salt accumulation from the caustic soda addition clearly limits the number of coagu-lation/redispersion cycles. This led Zhu and co-workers [ 71 ]to turn their attention to another monomer, namely ami-dine (N-amidino)dodecyl acrylamide (Figure 9 b ), whichwas already employed by Yuan and co-workers [ 21 ] as aCO 2-responsive monomer. This resulted in PS latexes that showed reversible coagulation and redispersion properties. Simultaneous, bubbling with N 2 and heating induce latexes coagulation. Redispersion of the latexes is achieved by introduction of CO 2, which switch amidine moieties from a neutral to a charged state. This process could be repeated several times. Notable, with a higher amidine concentration, the PS latexes exhibit a better sta-bility against electrolytes.Figure 7. S witchable aggregation–redispersion of a PMMA latex by the stimulation of CO 2 using a surfactant on the basis of ami-dine. Reproduced with permission. [ 68 ] Copyright 2012, American Chemical Society.Figure 8.Synthesis of a CO 2 -responsive PS latex and its reversibleaggregation/redispersion upon removal or addition of CO 2.Repro-duced with permission. [ 69 ]Copyright 2012, American Chemical Society.Figure 9.Switchable amidine copolymers used in the preparation of PS latexes, respectively. Panels (a) and (b) were redrawn fromthe literature. [ 70, 71 ]Macromol. Rapid Commun. 2013, 34, 1118−1133© 2013 WILEY-VCH Verlag GmbH & Co. KGaA, Weinheim。
高分子材料英文论文翻译
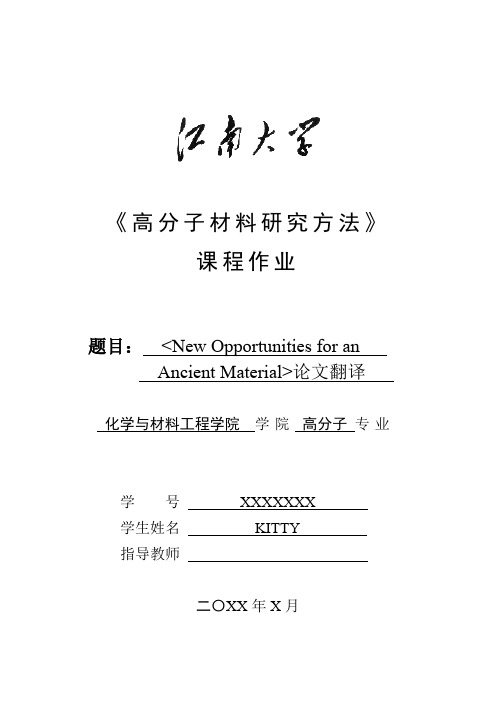
《高分子材料研究方法》课程作业题目:<New Opportunities for anAncientMaterial>论文翻译化学与材料工程学院学院高分子专业学号XXXXXXX学生姓名KITTY指导教师二〇XX年X月<New Opportunities for an Ancient Material>论文翻译Science 30 July 2010:V ol. 329 no. 5991 pp. 528-531DOI: 10.1126/science.1188936New Opportunities for an Ancient MaterialFiorenzo G. Omenetto, David L. Kaplan*Department of Biomedical Engineering, Tufts University, Medford, MA 02155, USA.E-mail: david.kaplan{at}一种古老材料的新机遇Fiorenzo G. Omenetto, David L. Kaplan*美国,马萨诸塞州(邮编02155),梅德福,塔夫茨大学,生物医学工程系邮箱:david.kaplan{at}ABSTRACTSpiders and silkworms generate silk protein fibers that embody strength and beauty. Orb webs are fascinating feats of bioengineering in nature, displaying magnificent architectures while providing essential survival utility for spiders. The unusual combination of high strength and extensibility is a characteristic unavailable to date in synthetic materials yet is attained in nature with a relatively simple protein processed from water. This biological template suggests new directions to emulate in the pursuit of new high-performance, multifunctional materials generated with a green chemistry and processing approach. These bio-inspired and high-technology materials can lead to multifunctional material platforms that integrate with living systems for medical materials and a host of other applications.摘要蜘蛛或桑蚕生成的丝蛋白纤维,是力与美的结合体。
- 1、下载文档前请自行甄别文档内容的完整性,平台不提供额外的编辑、内容补充、找答案等附加服务。
- 2、"仅部分预览"的文档,不可在线预览部分如存在完整性等问题,可反馈申请退款(可完整预览的文档不适用该条件!)。
- 3、如文档侵犯您的权益,请联系客服反馈,我们会尽快为您处理(人工客服工作时间:9:00-18:30)。
A. Lendlein, H. Jiang, O. Jü nger, R. Langer, Nature 2005, 434, 879
Example 3-- bioresponsive gels
PEG-based gels (a)crosslinked with peptides that are substrates for matrix metalloproteinases provide an environment for cell growth. Ingress of cells results in release of enzymes that can cleave specific crosslinks (b), which in turn can be used as a mechanism for release of further bioactive compounds such as growth factors, cell-signaling molecules, or drugs. M. P. Lutolf, J. L. Lauer-Fields, H. G. Schmoekel, A. T. Metters, F. E. Weber, G. B. Fields, J. A. Hubbell, Proc. Natl. Acad. Sci. USA 2003, 100, 5413.
cinnamic acid (CA) cinnamylidene acetic acid (CAA) n-butylacrylate (BA) hydroxyethyl methacrylate (HEMA) ethyleneglycol-1-acrylate-2-CA (HEA-CA)
Example 2—Polymer Characterization
Example 1—Polymer Characterization
K. Sumaru, M. Kameda, T. Kanamori, T. Shinbo, Macromolecules, 2004, 37, 4949.
Example 2—Shape memory polymer
A. Lendlein, H. Jiang, O. Jü nger, R. Langer, Nature 2005, 434, 879
Example 2—Shape memory polymer
A. Lendlein, H. Jiang, O. Jü nger, R. Langer, Nature 2005, 434, 879
Example 2—Polymer synthesis
poly(propylene glycol)-dimethacrylate (number average molecular mass Mn=560 g mol21, PPG2M560) as crosslinker. A. Lendlein, H. Jiang, O. Jü nger, R. Langer, Nature 2005, 434, 879
Example 3– Synthesis using Michael-type addition
J. Shaw et al. Proteomics 2003, 3, 1181–1195
M. P. Lutolf, J. L. Lauer-Fields, H. G. Schmoekel, A. T. Metters, F. E. Weber, G. B. Fields, J. A. Hubbell, Proc. Natl. Acad. Sci. USA 2003, 100, 5413.
(ii). Action of proteases that can cleave the peptide sequence (at sites A or B) removes protonated Arg resulting in collapse of the bead structure (iii) and reduced molecular accessibility of the hydrogel pores.
By Cameron Alexander* and Kevin M. Shakesheff
Division of Advanced Drug Delivery and Tissue Engineering School of Pharmacy, Boots Science Building University of Nottingham University Park, Nottingham NG7 2RD (UK)
Adv. Mater. 2006, 18, 3321–3328
Different types of stimulus response polymers
Light-Responsive Polymers Polymers Responsive to Biochemical Stimuli Thermal- and pH-Responsive Polymers Potential applications Responsive Polymers in Drug Delivery Responsive Polymers in Cell Culture and Regenerative Medicine Potential applications in Pre-clinical studies
Example 1—Polymer system
Photochemically switchable spirobenzopyran polymers as prepared by Sumaru et al., with proposed neutral, zwitterionic, and ionic states (I–IV).
K. Sumaru, M. Kameda, T. Kanamori, T. Shinbo, Macromolecules, 2004, 37, 4949.
Example 1—Polymer synthesis
K. Sumaru, M. Kameda, T. Kanamori, T. Shinbo, Macromolecules, 2004, 37, 4949.
Example 1—Polymer Characterization
K. Sumaru, M. Kameda, Tomolecules, 2004, 37, 4949.
Example 1—Polymer Characterization
K. Sumaru, M. Kameda, T. Kanamori, T. Shinbo, Macromolecules, 2004, 37, 4949.
A. Rehor, J. A. Hubbell, N. Tirelli, Langmuir 2005, 21, 411.
Example 5-- enzyme-responsive polymer hydrogels
Schematic description of enzymeresponsive polymer hydrogels. Polyacrylamide beads crosslinked with poly(ethylene glycol) (PEGA800) and pendant primary amine functionality are derivatised with enzyme-cleavable peptide linkers. Peptides contained an enzyme-cleavable section consisting of Gly (R =H) or Phe (R = CH2–C6H5) and an Arg residue causing electrostatic repulsion of polymer chains and swelling of the beads
Example 1—Polymer Characterization
K. Sumaru, M. Kameda, T. Kanamori, T. Shinbo, Macromolecules, 2004, 37, 4949.
Example 1—Polymer Characterization
K. Sumaru, M. Kameda, T. Kanamori, T. Shinbo, Macromolecules, 2004, 37, 4949.
Organic Division Seminar
Responsive Polymers at the Biology/Materials Science Interface
Advisor: Dr. Ken D. Shimizu Reviewed by Yagang Zhang 03-10-2008
Responsive Polymers at the Biology and Materials Science Interface
Example 3-- bioresponsive gels-synthesis
HFFs-Human doreskin fibroblasts MMPs-metalloproteinases
Substrate activity Adhesion ligand concentration Network crosslinking density Cell culture study Inverted phasecontrast microscopy Confocal scanning laser microscopy Bone healing in the rat cranium model M. P. Lutolf, J. L. Lauer-Fields, H. G. Schmoekel, A. T. Metters, F. E. Weber, G. B. Fields, J. A. Hubbell, Proc. Natl. Acad. Sci. USA 2003, 100, 5413.