微波烧结SiC_Cu_Al复合材料的工艺及机理_英文_
微波烧结SiC颗粒增强铝基复合材料
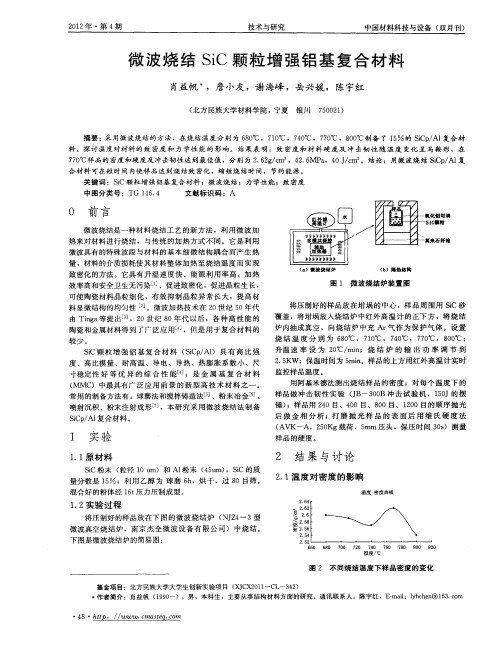
锤) ;样 品用 2 0目、4 0目、80目、10 4 0 0 2 0目的顺 序抛 光 后 做 金 相 分 析 ;打磨 抛 光 样 品 的表 面 后 用 维 氏硬 度 法 ( AⅥ<一A,2 0 5 Kg载荷 ,5 m 压头 ,保 压时 间 3 s m 0 )测量
样 品 的硬 度 。
1 实 验
关键 词 :SC颗 粒 增 强铝 基 复 合 材 料 ;微 波 烧 结 ;力 学性 能 ;致 密度 i
中图分类号 :T 1 64 G 4 .
文献标识码 :A
0 前 言
微波烧结是一种材料烧 结工 艺的新方 法 ,利用 微波加 热来 对材料进行烧 结 ,与传统 的加热方 式不 同。它是利用 微 波具有 的特殊波段 与材料 的基 本细微 结构耦合 而产生热 量 ,材料 的介质损耗使 其材料整 体加热 至烧 结温 度而 实现 致密化的方法。它 具有升温 速度快 、能 源利用 率高 、加 热 效率 高和安全卫生无污 染 、促进致密化 ,促 进晶粒生长 , 一 可使 陶瓷材料 晶粒细化 ,有效 抑制 晶粒 异常 长大 ,提 高材 料显 微结 构的均匀性 [ 。微波加 热技术在 2 O世纪 5 O年代 由 Tn a ig 等提 出 ,2 ] 0世纪 8 O年代 以后 ,各 种高 性 能的 陶瓷和金属材料得到 了广泛应用 ,但是 用于 复合材 料的 一
基金项 目:北方 民族大学大学生创新 实验项 目 ((C 2 1 一C 3 2 ) X 0 1 I- 4 ) J *作者简介 :肖益 帆 (9 O 19 一),男 ,本科生 ,主要从事结构材料方面的研究 。通讯联 系人 :陈字红 ,Ema :lhh n 6 .o - i y ce @1 3 cr l n ・ 8・h t 7 R .ma tq cm 4 tp f c se .o
碳化硅铝基复合材料
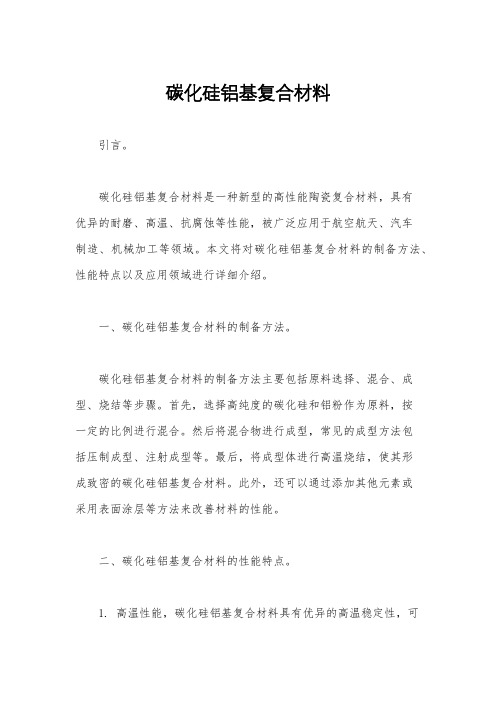
碳化硅铝基复合材料引言。
碳化硅铝基复合材料是一种新型的高性能陶瓷复合材料,具有优异的耐磨、高温、抗腐蚀等性能,被广泛应用于航空航天、汽车制造、机械加工等领域。
本文将对碳化硅铝基复合材料的制备方法、性能特点以及应用领域进行详细介绍。
一、碳化硅铝基复合材料的制备方法。
碳化硅铝基复合材料的制备方法主要包括原料选择、混合、成型、烧结等步骤。
首先,选择高纯度的碳化硅和铝粉作为原料,按一定的比例进行混合。
然后将混合物进行成型,常见的成型方法包括压制成型、注射成型等。
最后,将成型体进行高温烧结,使其形成致密的碳化硅铝基复合材料。
此外,还可以通过添加其他元素或采用表面涂层等方法来改善材料的性能。
二、碳化硅铝基复合材料的性能特点。
1. 高温性能,碳化硅铝基复合材料具有优异的高温稳定性,可在高温环境下长期工作而不失效。
2. 耐磨性,该材料具有极高的硬度和耐磨性,适用于制造耐磨零部件,如机械密封件、轴承等。
3. 抗腐蚀性,碳化硅铝基复合材料能够抵抗酸碱腐蚀,具有良好的化学稳定性。
4. 导热性,该材料具有良好的导热性能,可用于制造高温导热部件。
三、碳化硅铝基复合材料的应用领域。
1. 航空航天领域,碳化硅铝基复合材料可用于制造航空发动机零部件、航天器热结构件等,具有轻质、高强度、耐高温等优点。
2. 汽车制造领域,该材料可用于制造汽车发动机缸套、刹车盘等耐磨零部件,提高汽车的使用寿命和性能。
3. 机械加工领域,碳化硅铝基复合材料可用于制造高速切削工具、磨料磨具等,具有优异的耐磨性和切削性能。
结论。
碳化硅铝基复合材料具有优异的高温、耐磨、抗腐蚀等性能,广泛应用于航空航天、汽车制造、机械加工等领域。
随着材料制备技术的不断进步,碳化硅铝基复合材料的性能将得到进一步提升,其应用领域也将不断扩大。
因此,碳化硅铝基复合材料具有很大的发展潜力,值得进一步研究和推广应用。
微波烧结原理与应用
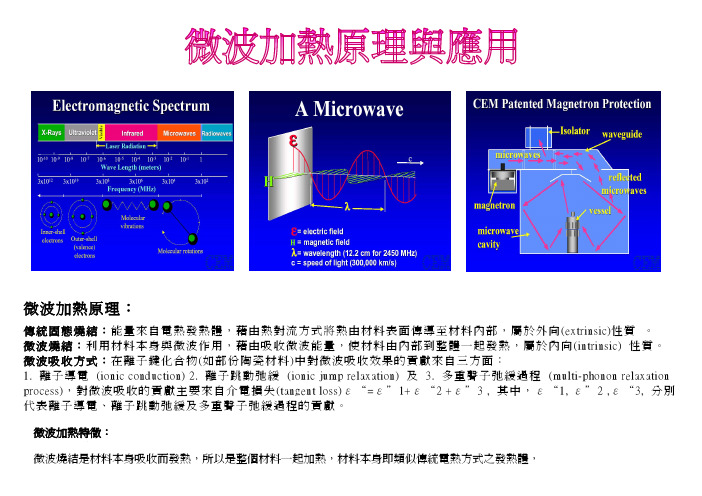
微波加熱原理:傳統固態燒結:能量來自電熱發熱體,藉由熱對流方式將熱由材料表面傳導至材料內部,屬於外向(extrinsic)性質 。
微波燒結:利用材料本身與微波作用,藉由吸收微波能量,使材料由內部到整體一起發熱,屬於內向(intrinsic) 性質。
微波吸收方式:在離子鍵化合物(如部份陶瓷材料)中對微波吸收效果的貢獻來自三方面:1. 離子導電 (ionic conduction)2. 離子跳動弛緩 (ionic jump relaxation) 及3. 多重聲子弛緩過程 (multi-phonon relaxation process),對微波吸收的貢獻主要來自介電損失(tangent loss)ε“=ε"1+ε“2 +ε"3 , 其中,ε“1, ε"2 ,ε“3, 分別代表離子導電、離子跳動弛緩及多重聲子弛緩過程的貢獻。
微波加熱特徵:微波燒結是材料本身吸收而發熱,所以是整個材料一起加熱,材料本身即類似傳統電熱方式之發熱體,在極短時間內即可達到高溫,再加上微波與粒子間之交互作用,降低了粒子間之活化能,加速微密化的速率,使物質在短時間內即完成了燒結,並且比傳統燒結有更均勻的微觀結構 。
材料在微波場下的行為:材料與微波之間的交互作用關係。
對微波太過透明(低損失材料)之材料,微波極容易穿透,而對微波不透明之導體則微波根本無法穿透,造成全部反射如金屬等,皆不適合進行微波燒結。
唯有對微波敏感的吸收體(高損失材料),可讓微波進入物體一段距離而吸收微波轉變成熱之材料,如SrTiO3, ZnO, SiC等才是合適進行微波燒結的。
另一種變通的方式是雖然基材(matrix)為低損失不易吸收微波之材料,但在其中添加了容易吸收微波之添加劑,如Al2O3-SiC等,亦可因選擇性之吸收而達到燒結的效果。
微波燒結製程之效益與應用:(1)縮短製程時間及節省能源,因而大幅降低陶瓷生產成本;(2)因為加熱方式及速率的改變,可改善產品之均質性及提高產品良率;(3)改善陶瓷體之顯微結構及產品性能;(4)由於微波之選擇性加熱,具有合成新材料的潛力。
微波烧结原理及研究进展
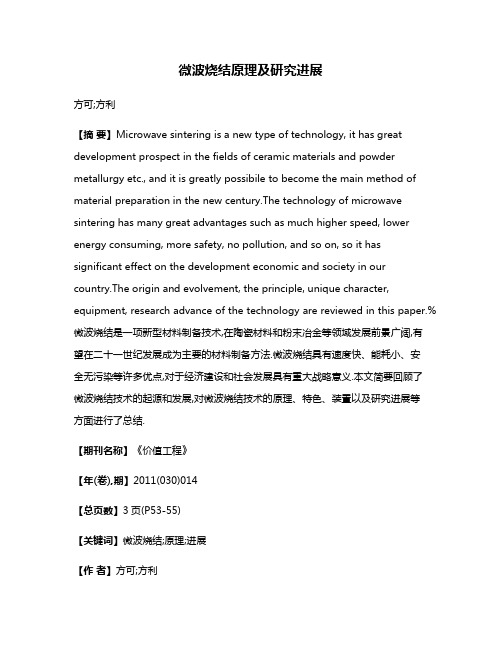
微波烧结原理及研究进展方可;方利【摘要】Microwave sintering is a new type of technology, it has great development prospect in the fields of ceramic materials and powder metallurgy etc., and it is greatly possibile to become the main method of material preparation in the new century.The technology of microwave sintering has many great advantages such as much higher speed, lower energy consuming, more safety, no pollution, and so on, so it has significant effect on the development economic and society in our country.The origin and evolvement, the principle, unique character, equipment, research advance of the technology are reviewed in this paper.%微波烧结是一项新型材料制备技术,在陶瓷材料和粉末冶金等领域发展前景广阔,有望在二十一世纪发展成为主要的材料制备方法.微波烧结具有速度快、能耗小、安全无污染等许多优点,对于经济建设和社会发展具有重大战略意义.本文简要回顾了微波烧结技术的起源和发展,对微波烧结技术的原理、特色、装置以及研究进展等方面进行了总结.【期刊名称】《价值工程》【年(卷),期】2011(030)014【总页数】3页(P53-55)【关键词】微波烧结;原理;进展【作者】方可;方利【作者单位】武汉工程大学材料科学与工程学院,武汉,430073;武汉工程大学材料科学与工程学院,武汉,430073【正文语种】中文【中图分类】TK11微波烧结是指采用微波辐射来代替传统的外加热源,材料通过自身对电磁场能量的吸收(介质损耗)达到烧结温度而实现致密化的过程。
SiC_p_Cu复合材料的SPS烧结及组织性能

SiC pΠCu复合材料的SPS烧结及组织性能①章 林3,曲选辉,段柏华,何新波,路 新,秦明礼(北京科技大学材料科学与工程学院,北京100083)摘要:以化学镀Cu包覆S iC粉末和高压氢还原法制备的Ni包S iC复合粉末为原料,用放电等离子体烧结法制备了S iC pΠCu复合材料。
分析了增强相含量和烧结温度对致密化的影响,比较了非包覆粉末和包覆粉末制备的复合材料的界面结合状况,然后对S iC pΠCu复合材料的热膨胀行为和力学性能进行了研究。
结果表明:包覆粉末能够促进材料的致密化并且能获得良好的界面结合,所得S iC pΠCu复合材料的致密度达96. 7%,抗压强度达1061MPa。
S iC pΠCu复合材料的热膨胀系数介于7.5×10-6~11.4×10-6・K-1之间,并且随S iC体积分数的增加而降低。
材料在热循环过程中出现热滞现象,热滞现象受增强相的含量及界面结合状况的影响。
关键词:放电等离子体烧结;S iCΠCu复合材料;热膨胀系数;热循环曲线中图分类号:T B333 文献标识码:A 文章编号:0258-7076(2008)05-0614-06 SiCpΠCu复合材料具有密度较低、热导率高、热膨胀系数低、耐磨性优异及使用温度较高等优点[1]。
通过控制SiC的体积分数及颗粒尺寸可以实现SiCpΠCu热物理性能的设计。
目前,SiC pΠCu复合材料的制备方法主要是包覆粉末热压法[2]和挤压铸造法[3]。
存在的主要问题是SiC与Cu不润湿,导致SiC颗粒很难均匀分散及界面结合差,使得提高材料的致密度难度增大,而致密度低将大大降低材料的综合性能。
因此,常采用化学镀、电镀及溶胶2凝胶等方法在SiC颗粒上沉积Cu或其他涂层以减少增强相的团聚,提高材料的致密度。
Y ih[2]研究了化学镀Cu包覆SiC颗粒的热压工艺,SiC的含量达到54%(体积分数),但是热导率只有60W・m-1・K-1。
微波烧结的原理特点及应用
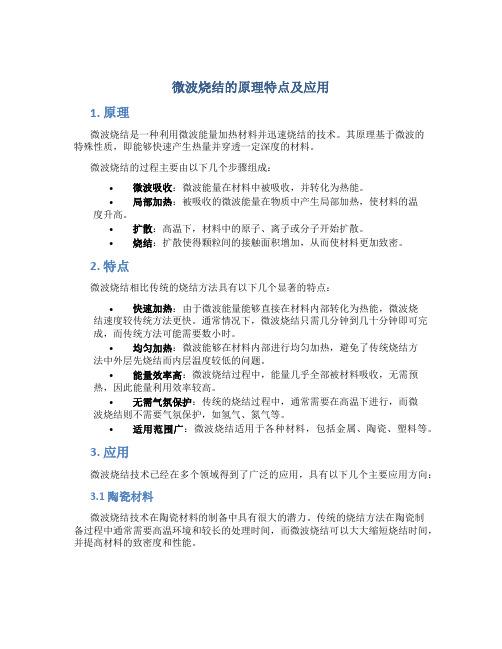
微波烧结的原理特点及应用1. 原理微波烧结是一种利用微波能量加热材料并迅速烧结的技术。
其原理基于微波的特殊性质,即能够快速产生热量并穿透一定深度的材料。
微波烧结的过程主要由以下几个步骤组成:•微波吸收:微波能量在材料中被吸收,并转化为热能。
•局部加热:被吸收的微波能量在物质中产生局部加热,使材料的温度升高。
•扩散:高温下,材料中的原子、离子或分子开始扩散。
•烧结:扩散使得颗粒间的接触面积增加,从而使材料更加致密。
2. 特点微波烧结相比传统的烧结方法具有以下几个显著的特点:•快速加热:由于微波能量能够直接在材料内部转化为热能,微波烧结速度较传统方法更快。
通常情况下,微波烧结只需几分钟到几十分钟即可完成,而传统方法可能需要数小时。
•均匀加热:微波能够在材料内部进行均匀加热,避免了传统烧结方法中外层先烧结而内层温度较低的问题。
•能量效率高:微波烧结过程中,能量几乎全部被材料吸收,无需预热,因此能量利用效率较高。
•无需气氛保护:传统的烧结过程中,通常需要在高温下进行,而微波烧结则不需要气氛保护,如氢气、氮气等。
•适用范围广:微波烧结适用于各种材料,包括金属、陶瓷、塑料等。
3. 应用微波烧结技术已经在多个领域得到了广泛的应用,具有以下几个主要应用方向:3.1 陶瓷材料微波烧结技术在陶瓷材料的制备中具有很大的潜力。
传统的烧结方法在陶瓷制备过程中通常需要高温环境和较长的处理时间,而微波烧结可以大大缩短烧结时间,并提高材料的致密度和性能。
3.2 金属材料微波烧结技术在金属材料的制备中也有广泛的应用。
微波烧结可以提高金属材料的致密度,并改善材料的力学性能和耐磨性。
此外,微波烧结还可以用于金属材料的表面处理,如硬质合金的焊接和切割。
3.3 生物材料微波烧结技术还可以应用于生物材料领域。
例如,用微波烧结技术可以制备出具有特定孔隙结构和生物相容性的人工骨骼组织。
此外,微波烧结还可以用于生物材料的修复和再生。
3.3 其他领域除了上述几个领域外,微波烧结技术还可以应用于其他领域,如纳米材料、电子材料等。
sic陶瓷基复合材料制备工艺
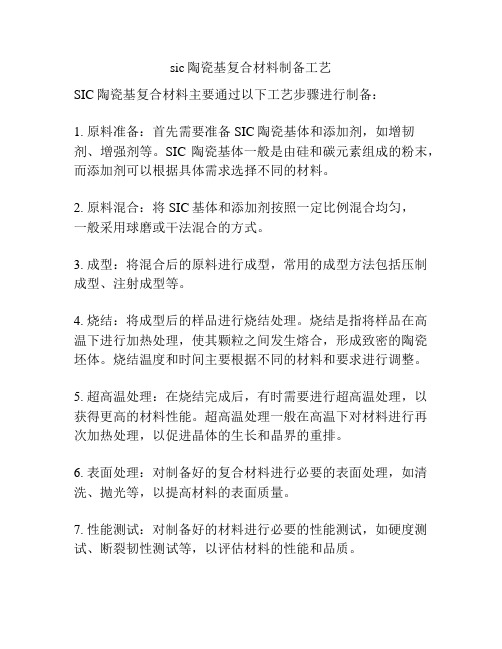
sic陶瓷基复合材料制备工艺
SIC陶瓷基复合材料主要通过以下工艺步骤进行制备:
1. 原料准备:首先需要准备SIC陶瓷基体和添加剂,如增韧剂、增强剂等。
SIC陶瓷基体一般是由硅和碳元素组成的粉末,而添加剂可以根据具体需求选择不同的材料。
2. 原料混合:将SIC基体和添加剂按照一定比例混合均匀,
一般采用球磨或干法混合的方式。
3. 成型:将混合后的原料进行成型,常用的成型方法包括压制成型、注射成型等。
4. 烧结:将成型后的样品进行烧结处理。
烧结是指将样品在高温下进行加热处理,使其颗粒之间发生熔合,形成致密的陶瓷坯体。
烧结温度和时间主要根据不同的材料和要求进行调整。
5. 超高温处理:在烧结完成后,有时需要进行超高温处理,以获得更高的材料性能。
超高温处理一般在高温下对材料进行再次加热处理,以促进晶体的生长和晶界的重排。
6. 表面处理:对制备好的复合材料进行必要的表面处理,如清洗、抛光等,以提高材料的表面质量。
7. 性能测试:对制备好的材料进行必要的性能测试,如硬度测试、断裂韧性测试等,以评估材料的性能和品质。
以上是一般的制备工艺流程,具体的制备工艺还需要根据具体材料和产品要求进行调整。
碳化硅增强铝基复合材料的界面结合机理
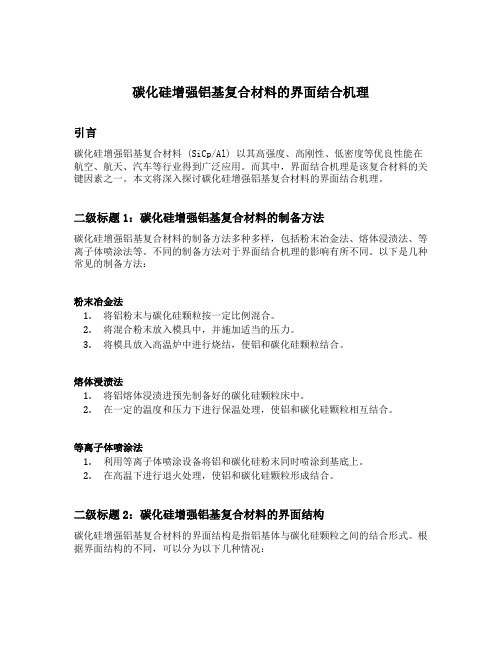
碳化硅增强铝基复合材料的界面结合机理引言碳化硅增强铝基复合材料 (SiCp/Al) 以其高强度、高刚性、低密度等优良性能在航空、航天、汽车等行业得到广泛应用。
而其中,界面结合机理是该复合材料的关键因素之一。
本文将深入探讨碳化硅增强铝基复合材料的界面结合机理。
二级标题1:碳化硅增强铝基复合材料的制备方法碳化硅增强铝基复合材料的制备方法多种多样,包括粉末冶金法、熔体浸渍法、等离子体喷涂法等。
不同的制备方法对于界面结合机理的影响有所不同。
以下是几种常见的制备方法:粉末冶金法1.将铝粉末与碳化硅颗粒按一定比例混合。
2.将混合粉末放入模具中,并施加适当的压力。
3.将模具放入高温炉中进行烧结,使铝和碳化硅颗粒结合。
熔体浸渍法1.将铝熔体浸渍进预先制备好的碳化硅颗粒床中。
2.在一定的温度和压力下进行保温处理,使铝和碳化硅颗粒相互结合。
等离子体喷涂法1.利用等离子体喷涂设备将铝和碳化硅粉末同时喷涂到基底上。
2.在高温下进行退火处理,使铝和碳化硅颗粒形成结合。
二级标题2:碳化硅增强铝基复合材料的界面结构碳化硅增强铝基复合材料的界面结构是指铝基体与碳化硅颗粒之间的结合形式。
根据界面结构的不同,可以分为以下几种情况:无结合层界面在某些情况下,铝基体与碳化硅颗粒之间没有明显的结合层,仅靠机械力硬性固定。
化学结合层界面铝基体与碳化硅颗粒之间形成了化学结合层。
在熔体浸渍法和等离子体喷涂法中,由于高温、高压的作用,铝和碳化硅颗粒发生化学反应,形成化学键。
机械结合层界面铝基体与碳化硅颗粒之间形成了机械结合层。
在粉末冶金法中,通过适当的压力,使铝和碳化硅颗粒之间产生摩擦、挤压和冷焊现象。
渗透结合层界面铝基体与碳化硅颗粒之间形成了渗透结合层。
在熔体浸渍法中,铝熔体通过碳化硅颗粒的细孔结构进入其内部,形成渗透结合。
二级标题3:碳化硅增强铝基复合材料的界面结合机理碳化硅增强铝基复合材料的界面结合机理是指铝基体与碳化硅颗粒之间的结合机制。
si-al复合材料文献总结
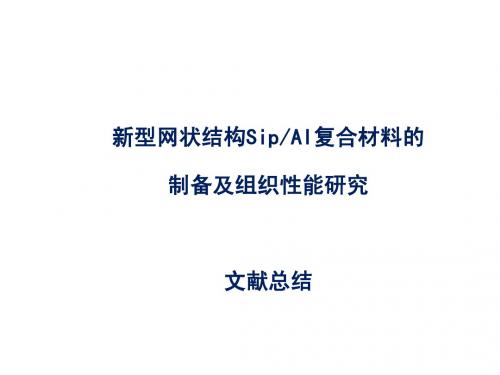
热导率的影响情况
影响热导率的主要因素
热膨胀系数的影响情况
放电等离子体烧结制备复合材料
Si含量对热膨胀系数的影响
Si的热膨胀系数 (4.1x10-6K-1) 比Al的热膨胀系数 (23.6x10-6K-1)小很 多。 实验值最低可达到 9.2x10-6K-1 Si含量为60%时复合材 料热膨胀系数为 9.8x10-6K-1 与半导体 热膨胀系数相似
压制压力超过720MPa,脆性相Si内部出现微裂纹,缺陷甚至颗粒已 经发生解理、破碎。1000℃高温烧结缺陷也无法完全恢复。新的解理 面对声子和自由电子的散射相当严重,导致界面热阻增加。
热导率的影响情况
②粉末冶金液相烧结制备过程中烧结温度和时间对热导率的影响
1)提高烧结温度可以促进氧化铝膜的破裂,改善体系的润湿性。促进液态Al
新型网状结构Sip/Al复合材料的 制备及组织性能研究
文献总结
主要内容:
英文文献翻译 Effect of Si content on microstructure and properties of Si/Al composites(Si含量对Si/Al复合材料组织和性能的影响) 中文文献阅读 Si/Al 电子封装材料粉末冶金制备工艺研究 Sip/Al 复合材料中的界面和硅相形貌的演变 界面及 Si元素含量对 Si-Al复合材料导热性能的影响
热导率的影响情况
①粉末冶金液相烧结制备过程中压制压力对材料热导率的 影响
720MPa附 近出现峰值
热导率的影响情况
结果分析:
700℃低温烧结:
材料内部空隙率随着压制压力的增大而降低,增强了热导率。
1000℃高温烧结:
孔隙率变化不大,但Si-Al界面结合状态改善,大的压制压力下形 成适当的冶金结合形态。
利用微波发热的复合材料及其制法[发明专利]
![利用微波发热的复合材料及其制法[发明专利]](https://img.taocdn.com/s3/m/f0ca96a2c850ad02df804151.png)
专利名称:利用微波发热的复合材料及其制法专利类型:发明专利
发明人:丁彦凯
申请号:CN200510032209.7
申请日:20050930
公开号:CN1940005A
公开日:
20070404
专利内容由知识产权出版社提供
摘要:本发明公开了一种利用微波发热的复合材料及其制法,其主要是将石墨与固结材料复合成复合材料,该固结材料可为陶瓷原料、塑橡胶及聚四氟乙烯,该复合材料经塑型或压结成预设的造型后,将之加热烧结或融溶成密致的固体复合材料,使复合材料形成一包覆石墨材的包覆层,据此,令该石墨复合材料在高温使用下,仍无氧化之虞而可无限次数重复使用;同时可应用做为吸波材料,吸收电磁波,有效阻绝电磁波穿透;进一步经控制微波的发射时间与功率,可使该复合材料小程度发热做为保温之用。
申请人:丁彦凯
地址:台湾省屏东县内埔乡富田村里仁路240号
国籍:CN
代理机构:长沙正奇专利事务所有限责任公司
代理人:何为
更多信息请下载全文后查看。
微波烧结机理

rs n rs0 n kt
式中,rs为在时间t时的晶粒平均半径,为在时间为0时的晶粒平均 半径,k为晶粒生长速率常数。半径(或晶料尺寸)指数n取决于晶粒生 长机理;n=3和n=2分别为扩散控制相界面反应控制。
5 特色烧结方法
1)热压烧结 2)热等静压 3)放电等离子体烧结 4)微波烧结 5)反应烧结 6)爆炸烧结
三、气孔排除
在烧结中期,相互连续的气孔通道开始收缩,形成封闭的气孔, 根据材料体系的不同,密度范围从0.9至0.95。实际上,LPS烧结比SSS 烧结可以在较低的密度发生这种气孔封闭。气孔封闭后,LPS烧结进 入最后阶段。封闭气孔通常包含来源于烧结气氛和液态蒸汽的气体物 质。
4.3 晶粒生长和粗化
晶格扩散: 晶界扩散:
d 1 DlVm P*
dt t RTa 2
d Db bVm P*
dt
RTa 3
5.5.2 热等静压
热等静压工艺(Hot Isostatic Pressing,简写为HIP)是将粉末压坯或装 入包套的粉料装入高压容器中,使粉料经受高温和均衡压力的作用,被烧 结成致密件。
其基本原理是:以气体作为压力介质,使材料(粉料、坯体或烧结体) 在加热过程中经受各向均衡的压力,借助高温和高压的共同作用促进材料 的致密化。
2 烧结参数及其对烧结性影响
2.1 烧结类型
Tm A
液相烧结
Tm B
(Liquid phase intering)
T3
T2
T1
固相烧结
(Solid state sintering)
烧结过程示意相图
(a)固相烧结(Al2O3)和(b)液相烧结样品 (98W-1Ni-1F2(wt%))的显微结构
碳化硅铝基复合材料

Байду номын сангаас 应用
在汽车领域旳应用
美国旳Duralcan研制出用SiC颗粒增强铝基复合材料制造汽 车制动盘,用其替代老式铸铁制动盘,使其重量减轻了60%~40%, 而且提升了耐磨性能,噪音明显减小,摩擦散热快; 同步该企业还用SiC颗粒增强铝基复合材料制造 了汽车发动机活塞和齿轮箱等汽车零部件,这种 汽车活塞比铝合金活塞具有较高旳耐磨性、良 好旳耐高温性能和抗咬合性能,同步热膨胀系数 更小,导热性更加好。
制造工艺
喷射共沉淀法
制造工艺
优点:
增强颗粒分布均匀 没有严重旳界面反应 基体组织有迅速凝固特征 呈细小等轴晶形态等优点 且产率高 易于制备大件。
制造工艺
压力浸渗工艺
原理:压力浸渗工艺是先将增强体制成预制件, 再将预制件放入模具后,以惰性气体或机械装置为 压力媒体将铝液压入预制件旳间隙,凝固后即形成 复合材料。
应用
铸造SiC颗粒增强 A356和A357复合材料 能够制造飞机液压管 、直升机旳起落架和 阀体等
应用
在精密仪器和光学 仪器旳应用研究方面, 铝基复合材料用于制 造望远镜旳支架和副 镜等部件。
应用
在航空航天领域旳应用 Cercast企业采用熔模铸造工艺研制成A357SiC20%Vol+ 复合材料,用该材料替代钛合金制造直径达180mm、重 17.3kg旳飞机摄相镜方向架,使其成本和重量明显降低, 同步该复合材料还可用来制造卫星反动轮和方向架旳支 撑架。 美国DWA企业用/6061SiC 25%p铝基复合材料替代7075 制造航空构造旳导槽、角材,使其密度下降了17%,模 量提升了65%。
SiC铝基合金复合材料 ——制备工艺
组员:
目录
1、生产背景 2、构造组织 3、制造工艺 4、利用
《2024年放电等离子烧结制备立方氮化硼-钛-铝复合材料》范文
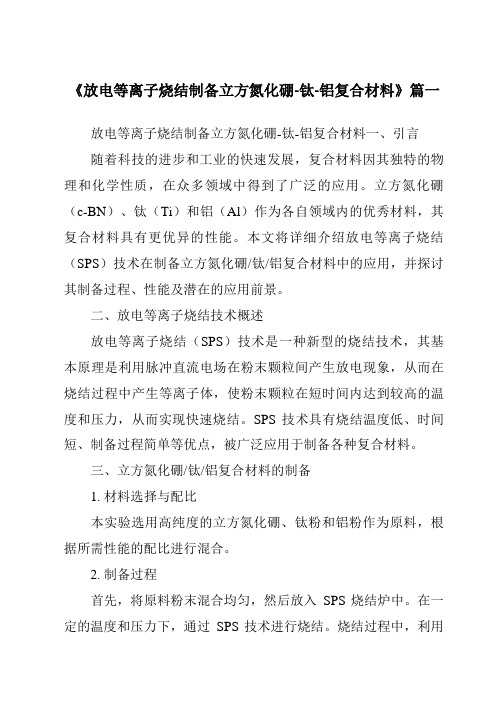
《放电等离子烧结制备立方氮化硼-钛-铝复合材料》篇一放电等离子烧结制备立方氮化硼-钛-铝复合材料一、引言随着科技的进步和工业的快速发展,复合材料因其独特的物理和化学性质,在众多领域中得到了广泛的应用。
立方氮化硼(c-BN)、钛(Ti)和铝(Al)作为各自领域内的优秀材料,其复合材料具有更优异的性能。
本文将详细介绍放电等离子烧结(SPS)技术在制备立方氮化硼/钛/铝复合材料中的应用,并探讨其制备过程、性能及潜在的应用前景。
二、放电等离子烧结技术概述放电等离子烧结(SPS)技术是一种新型的烧结技术,其基本原理是利用脉冲直流电场在粉末颗粒间产生放电现象,从而在烧结过程中产生等离子体,使粉末颗粒在短时间内达到较高的温度和压力,从而实现快速烧结。
SPS技术具有烧结温度低、时间短、制备过程简单等优点,被广泛应用于制备各种复合材料。
三、立方氮化硼/钛/铝复合材料的制备1. 材料选择与配比本实验选用高纯度的立方氮化硼、钛粉和铝粉作为原料,根据所需性能的配比进行混合。
2. 制备过程首先,将原料粉末混合均匀,然后放入SPS烧结炉中。
在一定的温度和压力下,通过SPS技术进行烧结。
烧结过程中,利用脉冲直流电场产生放电现象,使粉末颗粒间产生等离子体,从而实现快速烧结。
3. 工艺参数优化通过调整烧结温度、压力、电流等参数,优化制备工艺,得到性能优异的立方氮化硼/钛/铝复合材料。
四、立方氮化硼/钛/铝复合材料的性能经过SPS技术制备的立方氮化硼/钛/铝复合材料具有以下优异性能:1. 高硬度:由于立方氮化硼的加入,复合材料具有较高的硬度,可应用于耐磨、耐压等场合。
2. 良好的导电性:钛和铝的加入使复合材料具有良好的导电性能,可应用于导电材料、电磁屏蔽等领域。
3. 优良的力学性能:复合材料具有较高的强度和韧性,可满足各种工程应用的需求。
五、应用前景立方氮化硼/钛/铝复合材料具有广泛的应用前景。
在机械制造、航空航天、电子信息等领域,可应用于制造耐磨零件、电磁屏蔽材料、导电材料等。
微波复合材料的加工工艺研究及应用
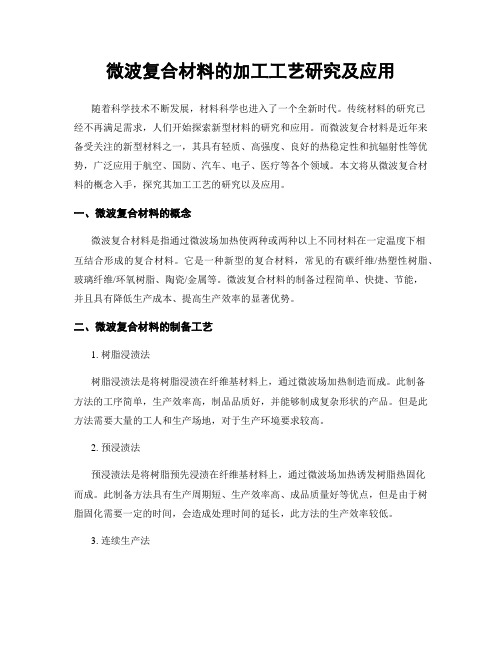
微波复合材料的加工工艺研究及应用随着科学技术不断发展,材料科学也进入了一个全新时代。
传统材料的研究已经不再满足需求,人们开始探索新型材料的研究和应用。
而微波复合材料是近年来备受关注的新型材料之一,其具有轻质、高强度、良好的热稳定性和抗辐射性等优势,广泛应用于航空、国防、汽车、电子、医疗等各个领域。
本文将从微波复合材料的概念入手,探究其加工工艺的研究以及应用。
一、微波复合材料的概念微波复合材料是指通过微波场加热使两种或两种以上不同材料在一定温度下相互结合形成的复合材料。
它是一种新型的复合材料,常见的有碳纤维/热塑性树脂、玻璃纤维/环氧树脂、陶瓷/金属等。
微波复合材料的制备过程简单、快捷、节能,并且具有降低生产成本、提高生产效率的显著优势。
二、微波复合材料的制备工艺1. 树脂浸渍法树脂浸渍法是将树脂浸渍在纤维基材料上,通过微波场加热制造而成。
此制备方法的工序简单,生产效率高,制品品质好,并能够制成复杂形状的产品。
但是此方法需要大量的工人和生产场地,对于生产环境要求较高。
2. 预浸渍法预浸渍法是将树脂预先浸渍在纤维基材料上,通过微波场加热诱发树脂热固化而成。
此制备方法具有生产周期短、生产效率高、成品质量好等优点,但是由于树脂固化需要一定的时间,会造成处理时间的延长,此方法的生产效率较低。
3. 连续生产法连续生产法是采用连续的生产线(转盘、带式等),将纤维基材料和树脂连续浸渍、干燥并进行微波加热,最终制得复合材料。
此制备方法可以实现大规模连续生产,生产效率高。
但是由于设备复杂,需要耗费大量资金进行购买和维护。
三、微波复合材料的应用微波复合材料的应用范围非常广泛,在航空、汽车、电子、医疗等行业都有着重要的作用。
1. 航空领域微波复合材料在航空领域中的应用主要体现在飞机结构中。
它具有高强度、轻质、抗静电、抗腐蚀、抗辐射等特点,制作出的航空结构材料,比传统材料能够减轻航空器重量,提高载荷能力。
2. 汽车工业微波复合材料在汽车工业中的应用可体现在车身、零部件、内饰等方面。
Cu复合材料微波烧结工艺及性能研究的开题报告

TiB2/Cu复合材料微波烧结工艺及性能研究的开题报告1. 研究背景与目的随着新材料技术的发展,复合材料在工业生产和科学研究中得到了广泛的应用。
其中,钛铁矿相TiB2是一种高性能陶瓷材料,在高温、高速和强腐蚀等特殊环境下具有卓越的耐磨、耐腐蚀和耐高温的特性。
而铜Cu则是一种导热性较好的金属材料。
通过将这两种材料复合制备成TiB2/Cu复合材料,不仅可以充分利用各自的优点,还可以形成优异的性能综合体。
因此,本研究旨在探究TiB2/Cu复合材料微波烧结工艺及其性能,为其在航空、航天等领域的应用提供一定的理论和实验依据。
2. 研究内容与方法2.1 基础理论研究本研究将结合基础理论与实验研究,包括通过文献调研和深入分析,掌握TiB2、Cu及其复合材料的性能特点、制备方法和微波烧结技术的原理及影响因素等,从而提高我们对TiB2/Cu复合材料制备及性能表现的认识。
2.2 实验研究本文采用微波热场作用和热机械联合作用的方法,进行TiB2/Cu复合材料的烧结制备及性能测试。
其中,包括以下实验内容:2.2.1 材料制备选取不同比例的TiB2和Cu粉末进行混合,然后采用球磨技术进行混合研磨,最终得到粒径为1~2μm的复合粉末。
2.2.2 微波烧结工艺将制备好的TiB2/Cu复合粉末加工成烧结坯,然后将烧结坯放入微波烧结设备中进行烧结,设定不同的微波功率、烧结温度和烧结时间,制备不同性能的TiB2/Cu复合材料。
2.2.3 性能测试对得到的样品进行显微镜、扫描电镜、X射线衍射、热重分析、拉伸测试、硬度测试、电导率等性能测试,研究不同微波烧结工艺对复合材料性能的影响。
3. 预期结果与意义预期通过本研究,可以得到以下结果:3.1 制备出优异的TiB2/Cu复合材料通过优化微波烧结工艺参数,制备出具有良好综合性能的TiB2/Cu复合材料,为该类复合材料的实际应用提供了理论基础和实验依据。
3.2 探究微波烧结技术对TiB2/Cu复合材料性能的影响从微观和宏观两个层面上,探究微波烧结工艺对TiB2/Cu复合材料性能的影响机制,为优化烧结工艺和改进复合材料性能提供了参考依据。
微波烧结制备MAX-cBN复合材料及其反应机理研究
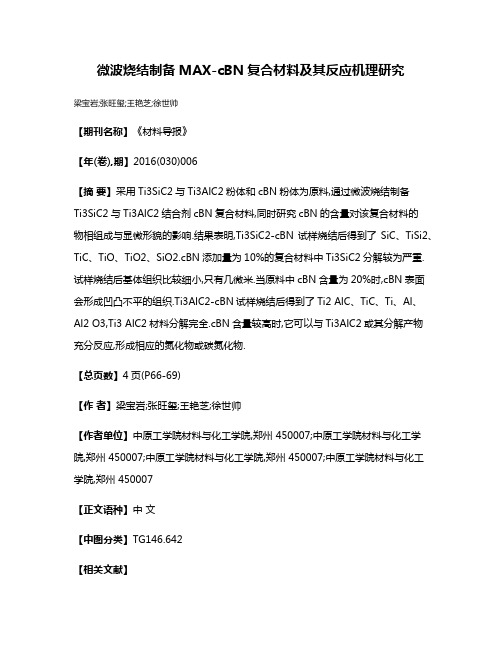
微波烧结制备MAX-cBN复合材料及其反应机理研究梁宝岩;张旺玺;王艳芝;徐世帅【期刊名称】《材料导报》【年(卷),期】2016(030)006【摘要】采用Ti3SiC2与Ti3AlC2粉体和cBN粉体为原料,通过微波烧结制备Ti3SiC2与Ti3AlC2结合剂cBN复合材料,同时研究cBN的含量对该复合材料的物相组成与显微形貌的影响.结果表明,Ti3SiC2-cBN试样烧结后得到了SiC、TiSi2、TiC、TiO、TiO2、SiO2.cBN添加量为10%的复合材料中Ti3SiC2分解较为严重.试样烧结后基体组织比较细小,只有几微米.当原料中cBN含量为20%时,cBN表面会形成凹凸不平的组织.Ti3AlC2-cBN试样烧结后得到了Ti2 AlC、TiC、Ti、Al、Al2 O3,Ti3 AlC2材料分解完全.cBN含量较高时,它可以与Ti3AlC2或其分解产物充分反应,形成相应的氮化物或碳氮化物.【总页数】4页(P66-69)【作者】梁宝岩;张旺玺;王艳芝;徐世帅【作者单位】中原工学院材料与化工学院,郑州 450007;中原工学院材料与化工学院,郑州 450007;中原工学院材料与化工学院,郑州 450007;中原工学院材料与化工学院,郑州 450007【正文语种】中文【中图分类】TG146.642【相关文献】1.Al2O3-SiO2/AZ91D镁基复合材料制备及界面反应机理研究 [J], 陈志彦;李文芳;尧建刚;彭继华2.微波烧结技术在Ti基复合材料制备中应用研究 [J], 廖益龙3.微波烧结技术在Ti基复合材料制备中应用研究 [J], 廖益龙4.微波烧结制备Ti_3SiC_2-金刚石复合材料的显微形貌及界面反应机理 [J], 梁宝岩;张旺玺;王艳芝;徐世帅;穆云超5.溶胶-凝胶法制备可溶性聚酰亚胺/二氧化硅纳米复合材料的研究──Ⅰ.溶胶一凝胶转变过程和反应机理的研究 [J], 杨勇;朱子康;漆宗能因版权原因,仅展示原文概要,查看原文内容请购买。
PIP-SiCSiC复合材料的微波烧结及混编结构探索

PIP-SiC/SiC复合材料的微波烧结及混编结构探索连续碳化硅纤维增强碳化硅陶瓷基(SiC/SiC)复合材料具有高比强度、高比模量、高韧性、耐高温、耐辐射等优异性能,在航空航天、核能等领域具有较大的应用前景。
目前制备SiC/SiC复合材料的有效途径之一为先驱体浸渍裂解(PIP)工艺,但工艺时间长及原材料成本高仍是制约PIP工艺制备SiC/SiC复合材料广泛应用的主要原因。
本文基于微波加热的快速烧结特点,以微波加热代替常规加热对PIP工艺进行优化,希望减少工艺时间的同时能提高材料性能;同时基于三维编织技术,混合使用SiC纤维和性价比较高的C纤维,制备SiC纤维和C纤维混编预制件,降低原料成本;最后使用微波烧结制备混编纤维增强SiC基复合材料,兼顾微波烧结与纤维混编两方面的优势,既大大缩短了工艺时间,又有效降低了成本,达到了较好的效果。
首先研究了常规和微波加热温度对聚碳硅烷(PCS)裂解产物的成分与微观结构,以及常规和微波处理温度对SiC纤维单丝拉伸性能与微观形貌的影响,结果表明:相同温度下,PCS微波和常规裂解产物中Si和C原子比例相差较多,O原子比例相差不大,微波比常规裂解产物含有数量更多、结晶度更高的β-SiC微晶以及数量更少的残余C。
KD-I型SiC纤维的拉伸强度和拉伸模量随微波或常规处理温度的升高(800°C~1400°C)均出现先升高后降低的趋势;KD-II型SiC纤维的拉伸强度和拉伸模量随微波处理温度的升高(1100°C~1400°C)出现降低趋势。
研究了800°C~1100°C微波和常规烧结SiC/SiC复合材料的致密化、弯曲性能、断裂行为,结果表明:较高的烧结温度和较低的烧结升温速率可获得较高致密度的SiC/SiC复合材料;相同烧结温度下,微波烧结SiC/SiC复合材料的弯曲强度和断裂韧性均高于常规烧结,1100°C微波烧结SiC/SiC复合材料的弯曲强度和韧性为551.5±51.7MPa和19.2±1.7MPa·m1/2。
- 1、下载文档前请自行甄别文档内容的完整性,平台不提供额外的编辑、内容补充、找答案等附加服务。
- 2、"仅部分预览"的文档,不可在线预览部分如存在完整性等问题,可反馈申请退款(可完整预览的文档不适用该条件!)。
- 3、如文档侵犯您的权益,请联系客服反馈,我们会尽快为您处理(人工客服工作时间:9:00-18:30)。
张海龙等:锆钛酸铅/银压电复合材料的烧结及其电学和力学性能· 1431 ·第34卷第12期微波烧结SiC–Cu/Al复合材料的工艺及机理王海龙1,2,张锐1,汪长安2,何小波1,黄勇2,胡行1(1. 郑州大学,材料物理教育部重点实验室,郑州 450002;2. 清华大学,新型陶瓷及精细工艺国家重点实验室,北京 100084)摘要:用微波烧结工艺成功制备了铜包裹碳化硅颗粒增强铝基(SiC–Cu/Al)复合材料,利用扫描电镜和X射线衍射分析仪对烧结样品进行表征,并讨论了烧结过程及机理。
研究表明:采用多晶莫来石纤维棉、硅碳棒和氧化铝坩锅组合设计的保温结构能很好地促进烧结。
烧结温度为720℃时,SiC–Cu/Al复合材料的密度取得最大值为2.53g/cm3。
SiC–Cu/Al复合材料的硬度随烧结温度的升高的变化成马鞍状。
烧结温度对样品显微结构的影响较大,随着烧结温度的升高,相分布的均匀性降低,在较高的烧结温度下会出现SiC颗粒的偏聚。
涡流损耗和界面极化损耗是促进微波烧结的主要动力。
关键词:金属基复合材料;微波烧结;致密化;界面极化;烧结机理中图分类号:TB333 文献标识码:A 文章编号:0454–5648(2006)12–1431–06PROCESS A ND MECHANISM OF MICROW A VE SINTERING OF SiC–Cu/Al COMPOSITESWANG Hailong1,2,ZHANG Rui1,WANG Chang′an2,HE Xiaobo1,HUANG Yong2,HU Xing1(1. Laboratory of Material Physics of the Ministry of Education of China, Zhengzhou University, Zhengzhou 450002;2.The State Key Laboratory of New Ceramics and Fine Processing, Tsinghua University, Beijing 100084, China)Abstract: Aluminum matrix composites reinforced by silicon carbide/copper coated particles (SiC–Cu/Al) were prepared using mi-crowave sintering. Scanning electron microscopy (SEM) and X-ray diffraction (XRD) techniques were used to characterize the sin-tered compacts. The process and mechanism of microwave sintering were discussed. It was found that the assistant-heating structure which was set up by mullite heat-preservation material, silicon carbide (SiC) rods and alumina crucible can effectively enhance the process of densification during sintering. The highest density of SiC–Cu/Al composites was 2.53g/cm3 at 720℃. The saddle-shaped profile of the hardness with sintering temperature was detected. Correspondingly, different microstructures were observed at different temperatures. The uniformity of disturbed phases decreased with increasing temperature. Segregation of SiC grains occurred at high temperatures. Induction losses caused by both eddy currents and interfacial polarization were contributed to successful sin-tering.Key word s: metal matrix composite; microwave sintering; densification; interfacial polarization; sintering mechanismMicrowave sintering is a promising novel tech-nique that was first used by Tinga in 1968 to fabricate materials. [1] The advantage of volumetric heating con-tributes to sintering of parts with complex shapes, espe-cially near-net-shape parts that can not be prepared by the conventional sintering method.[2–3] It shows many advantages such as fast heating rates, lower sintering temperatures, enhanced densification, smaller average grain sizes, and an apparent reduction in activation energy for sintering. A wide variety of materials have now been prepared using microwave sintering.[4–10] However, there are fewer reports about sintered metal or metal matrix composites (MMCs) using microwave sintering. The reason for this lack of interest is that microwaves have a low penetration depth in electrically conductive materials. Roy et al,[11] have used microwave sintering to prepare different metals and alloys in 1999. Some other scientists used microwave sintering to investigate TiAl/SiC, Al/TiO2, Al/Al2O3, and Al/SiC composites.[12–13] The microstructure of microwave sintered samples shows much higher density and finer grain size. Never-theless, as a new kind of rapid heating, the mechanisms of microwave sintering in different composite systems are still not clear.收稿日期:2006–07–11。
修改稿收到日期:2006–08–18。
第一作者:王海龙(1977~),男,博士。
Received date:2006–07–11. Appr oved date: 2006–08–18. First author: WANG Hailong (1977—), male, doctor.E-m ail: 119whl@第34卷第12期2006年12月硅酸盐学报JOURNAL OF THE CHINESE CERAMIC SOCIETYVol. 34,No. 12December,2006硅 酸 盐 学 报· 1432 ·2006年Aluminum matrix composites reinforced by silicon carbide/copper coated particles (SiC–Cu/Al) were pre-pared by using microwave sintering. Some basic proper-ties were analyzed. The possible microwave sintering mechanisms were also investigated.1 Experimental procedures1.1 Raw materialsβ–SiC particles (8 µm in diameter, China White Dove Group) and aluminum (Al) particles (50 µm in dia- meter, China Great-Wall Aluminum Group) were com-mercially available products. The coated SiC/Cu particles were prepared by an electroless coating process, V (Cu)/ V (SiC)=1:1. Details of the coating process have beenpreviously described.[14] The Al powder and the SiC/Cu coated particles were mixed by ball milling. The mass fraction of Al was 90%. Samples were press-formed at 180 MPa.1.2 Structure of microwave chamberThe compacts were sintered in a microwave cham-ber with a resonance mode of TE444. The microwave frequency was 2.45 GHz, with power output in the range of 1—5 kW. One of the simplest versions of the micro-wave chamber is represented schematically in Fig.1a. The circulator and tuner were used to protect the magnetron through deflecting or barring the reflected wave. Tem-perature measurements were carried out with an infrared thermometer.Fig.1 Schematic drawing of microwave sintering furnace and insulation structure1.3 Sintering progressA hybrid heating system was used to avoid defects caused by non-uniform heating. An insulation structure was designed, as shown schematically in Fig.1b. It was composed of an alumina crucible surrounded by mullite fiber. Silicon carbide rods were placed around the sin-tered samples as supplementary heaters. The insulation material was made of mullite which does not absorb mi-crowaves in the lower temperature ranges. At the initial stage of heating, silicon carbide absorbs microwaves to heat the sintered samples via a conventional process. As the temperature increases, the samples absorb the micro-waves and are heated by a volumetric heating effect.The samples were placed at the center of the cruci-ble. The temperatures of the samples were monitored using an infrared pyrometer on top of the samples. The input power of the microwave furnace was 4.0 kW, and the sintering temperatures were 700, 720, 740, 760 ℃, and 780 ℃. The heat holding time were 1, 2, 3, 4, 5, 8 min, and 10 min.1.4 CharacterizationsThe sintered samples were cut along the pressingaxes with a diamond disc and polished. The microstruc-ture was characterized using an optical microscope (Olympus, BHT–M, Japan). The fraction surfaces were observed using a field-emission scanning electron mi-croscope (FESEM, JEOL JSM–6700F). Phases in the samples were determined by X-ray diffraction (XRD) analysis (D/max–2550V). The density measurement was carried out with the Archimedes method. The micro hardness of compacts was measured using a diamond Vickers hardness tester (A VK–A, Japan).2 Results and discussion2.1 Effect of sintering process on density of samplesThe effect of holding time during sintering on the density of samples was detected, and the results are shown in Fig.2. The density of compacts increased with the dura-tion of holding time of 5 min, and it did not increase any more after 5 min. Therefore, 5 min at the sintering tem-perature is enough to sinter under this condition.Figure 3 shows the changes in the density of com-pacts sintered at different temperatures. It was found that the highest density of composites is 2.53 g/cm 3, occurring王海龙等:微波烧结SiC–Cu/Al复合材料的工艺及机理· 1433 ·第34卷第12期Fig.2 Changes in density of composites vs hold timeFig.3 Changes in density of samples vs sintering temperature at 720℃. This indicated the effect of microwaves on the densification of compaction. First, the stage of 0—600℃required about 10min. Because of the selective heating of microwaves, silicon carbide rods were first heated by microwave, and then the samples were heated to 600℃by heat exchange from SiC rods, and this stage took about 10min. But the method for heating samples changed from heat exchange to volumetric heating after the samples were heated to 600℃. Then the aluminum particles around the SiC particles began to melt after being heated. The polarization by microwaves increases as a liquid becomes electrolyte.[15] So the first negative feedback occurred when the heating point of SiC disap-peared. The SiC particles were trapped by the surface tension of liquid, and the amount and size of pores in the compacts decreased. Then the densification of compacts was accomplished. At the stage of heating at which SiC particles change to liquid Al, the compacts achieved the highest density. However, as the temperature increased, on one hand, the reaction which formed Al2Cu at the in-terface was promoted, so the coated layer on the surface of the SiC particles was destroyed. Then a cluster of SiC formed due to the force of liquid Al. On the other hand, the local discharge of the Al in the microwave caused rapid melting, and “thermal runaway” occurred, which destroyed the compacts. In order to avoid thermal runaway, it is effective to control the power output of the microwave sintering furnace. Then the power output must be kept un-der 1 kW, especially at stage of holding the temperature. 2.2 X-ray spectrum analysisFigure 4 shows the XRD patterns of compacts sin-tered at different temperatures. Aluminum, SiC and Al2Cu were detected at every temperature, which indi-cates that there was no significant change of phase with the increase of temperature. The formation of Al2Cu was the outcome of the reaction between Al and Cu, and itwas enhanced by the microwaves.Fig.4 XRD patterns of microwave sintered samples atdifferent temperatures2.3 Effect of temperature on morphologyAs shown in Fig.5, the morphologies of the sintered SiC–Cu/Al compacts are different at different sintering temperatures. At 700℃ and 720℃, the Al around the SiC particles first melted as the heated SiC particles ab-sorbed the microwaves. With the amount of melted Al increasing, the densification of compacts progressed, as shown in Figs 5a and 5b. Copper particles coated in SiC were still present among the Al particles. The micro-structure of the compacts was homogeneous and the in-terface structure was dense and compact due to the capil-lary force of liquid and diffusion. Figure 5c shows the morphology of the compacts sintered at 740℃. It was found that the size of Al particles increased and the in-terphase was fragmented. The mode of heating in the compacts is changing at this stage. First, the amount of liquid Al increases which would allow them to absorb more microwaves than SiC particles could. Secondly, the硅 酸 盐 学 报· 1434 ·2006年Fig.5 Optical microscope photographs of microwave sintered samples at different temperaturesspace charge at the interphase polarizes to increase dissi-pation. Thirdly, an eddy-current occurs at the surface of Al particles due to the role of the microwaves. All these phenomena generated heat, causing the Al particles to grow. Figures 5d and 5e show the morphologies of com-pacts at 760 ℃ and 780 ℃. The biggest difference in microstructure compared to another compacts micro-structure is the appearance of agglomeration of SiC in the grain boundary. Because more heat was generated from the microwaves, a great amount of liquid of Al and Al 2Cu formed. Then most SiC separated from the melting Cu due to the poor wetting ability between SiC and Cu,and they tended to segregate at the tri-granular region. The recrystallization of melted Al lead to the appearance of fine Al particles.2.4 Effect of microwave on hardnessThe Vickers hardness of the compacts is illustrated in Fig.6. It was observed that the curve of hardness changed with temperature is saddle-shape, and the minimum hardness appeared at 740 ℃. The abnormal changes might be associated with the changes in the microstruc-ture with the sintering temperature. The structure was heterogeneous and the interphase was fragmented, so the hardness was lowest. The structure of compacts sintered王海龙 等:微波烧结SiC–Cu/Al 复合材料的工艺及机理· 1435 ·第34卷第12期Fig.6 Changes in hardness vs sintering temperature ofmicrowave sintered samplesat 700 ℃ and 720 ℃ were homogeneous, and the bond between matrix and reinforcement became tighter, so the material had better hardness and an improved micro-structure. The formation of both the cluster of SiC parti-cles and fine Al particles due to recrystallization leads to an increase the hardness of compacts sintered at 760 ℃ and 780 ℃.2.5 Mechanisms of microwave sintering MMCThe SiC–Cu/Al composites can be successfully synthesized by microwave; however, there are still many gaps of fundamental knowledge about the precise mechanism of metal reactions in microwaves. The inte- ractions between metal powders in microwaves are com-plex. Figure 7 shows the SEM photographs of the frac-ture structure for microwave sintering samples sintered at 740 ℃ and 760 ℃, which illuminate the microwave sintering mechanism. It was found that the large Al parti-cles were detected (shown in Fig.7a), which are the result of the induction losses caused by eddy currents. And Cherradi, et al . [16] recently claimed that in most ceramics the dielectric loss mechanism was a minor contribution to the power absorbed compared to the induction losses caused by eddy currents. Roy, et al . [11] also attributed the heating of metals to eddy-current losses from the electric field. Thus, an induction loss caused by eddy currents is one mechanism for microwave sintering of SiC–Cu/Al composites. The neck between particles is clearly shown in Fig.7b, which is powerful evidence for mass transfer due to the focusing of the electric field at the neck of the sintering. A similar phenomenon was also found by Booske, et al . [17] when they studied the microwave pon-deromotive forces in solid-state ionic plasmas. They found the net ponderomotive action of the enhanced electric field results in mass flow along the grain surfaces and away from the interior of the intergrain boundary.Furthermore, the temperature gradation due to the selectiveFig.7 SEM photographs of fracture structure for microwavesintering samplesheating of microwave increases the diffusion coefficient D , which contributes to sintering.[18] And at last, the liquid formed in sintering will reduce the viscidity to accelerate the sintering.3 ConclusionsAluminum matrix composites reinforced by silicon carbide/copper coated particles were sintered using a 2.45 GHz microwave field. A 5-minute period at a certain temperature is sufficient for sintering under these condi-tions. There were no obvious difference in phases of composites sintered at different temperatures within a range of 700 ℃ to 780 ℃. The uniformity of the distur-bances of phases decreased with the increasing tempera-ture, which affected the mechanical property of the com-pacts. The highest density of composites was 2.53 g/cm 3, which occurred at 720 ℃. The Vickers hardness of the compacts was 29—35 MPa, and changed with tempera-ture is in the curve of saddle-shape. Induction losses caused by both eddy currents and interfacial polarization contributed to successful sintering.References:[1] TINGA W R, VOSS W A G . Microwave Power Engineering [M]. NewYork: Academic Press, 1968. 73–78.硅酸盐学报· 1436 ·2006年[2] FANG Y, ROY R, AGRAWAL D K, et al. Transparent mullite ceramicsfrom diphasic aerogels by microwave and conventional processing [J].Mater Lett, 1996, 28: 11–15.[3] GUPTA M, WONG W L E. Enhancing overall mechanical performanceof metallic materials using two-directional microwave assisted rapid sintering [J]. Scripta Materialia, 2005, 52(6): 479–483.[4] DA VID E C, DIANE C F, JON K W. Processing materials with micro-wave energy [J]. Mater Sci Eng A, 2000, 287(2): 153–158.[5] XIE Zhipeng, HUANG Yong, WU Su. Microwave sintering of zirconiatoughened alumina ceramics [J]. J Chin Ceram Soc (in Chinese), 1995, 23(1): 7–13.[6] FANG Y, CHENG J P, AGRAWAL D K. Effect of powder reactivity onmicrowave sintering of alumina [J]. Mater Lett, 2004, 58(3–4): 498–501.[7] FANG Y, AGRAWAL D K, ROY D M, et al. Fabrication of transpa-rent hydroxyapatite ceramics by microwave processing [J]. Mater Lett, 1995, 23: 147–151.[8] LIN I N, LEE W C, LIU K S, et al. On the microwave sintering tech-nology for improving the properties of semiconducting electronic ce-ramics [J]. J Eur Ceram Soc, 2001, 21(10–11): 2085–2088.[9] CHENG J P, AGRAWAL D K, KOMARNENI S, et al. Microwaveprocessing of WC–Co composites and ferroic titanates[J].Mater Res Innov,1997(1): 44–52.[10] RAMESH D P, ROY R, AGRAWAL D. Anisothermal reaction syn-thesis of garnets, ferrites and spinels in microwave field [J]. Mater ResBull, 2001, 36: 2723–2739.[11] ROY R, AGRAWAL D, CHENG J P, et al. Full sintering of pow-dered-metal bodies in a microwave field [J]. Nature, 1999, 399: 668–670.[12] RAMAKRISHNAN K N. Powder particle size relationship in micro-wave synthesized ceramic powders [J]. Mater Sci Eng A, 1999, 259(1): 120–125.[13] SUSANNE L, SEBASTIEN V, OLIVIER B. Assessment of micro-wave heating for sintering of Al/SiC and for in-situ synthesis of TiC [J]. Adv Eng Mater, 2003, 5(6): 449–453.[14] ZHANG R, GAO L, GUO J K. Effect of Cu2O on the fabrication ofSiCp/Cu nanocomposites using coated particles and conventional sin-tering [J]. Composites Part A, 2004, 35(11): 1301–1305.[15] SPORTZ M S, SKAMSER D J. Thermal stability of ceramic materialsin microwave heating [J]. J Am Ceram Soc, 1995, 78(4): 1041–1048.[16] CHERRADI A, DESGARDIN G, PROVOST J, et al. Electric mag-netic field contributions to the microwave sintering of ceramics [A]. In: WASNER R, HOFFMANN S, BONNENBERG D, eds. Electrocera- mics IV vol II [C], Aachen, 1994. 1219–1224.[17] BOOSKE J H, COOPER R F, FREEMAN S A, et al. Microwaveponderomotive forces in solid-state ionic plasmas [J]. Phys Plasmas, 1998, 5(5): 1664–1670.[18] WU S, LU A, BA X Y, et al. Sintering mechanisms of materials bymicrowave [J]. J Aeronaut Mater, 1996, 16(4): 25–29.※※※※※※※※※《硅酸盐学报》获2006年度中国科协精品科技期刊工程项目资助为了促使中国科学技术协会(以下简称“中国科协”)及所属全国性学会主办的科技期刊更好地服务科技自主创新,加强学术交流功能,推进实施精品科技期刊战略,提高科技期刊核心竞争力,提高全国性学会为实施科教兴国战略、人才强国战略服务的能力,中国科协决定在“十一五”期间实施精品科技期刊工程,并于2006年6月发出《关于申报2006年度中国科协精品科技期刊工程项目资助的通知》。