Gravitational wave
引力波探测及其意义
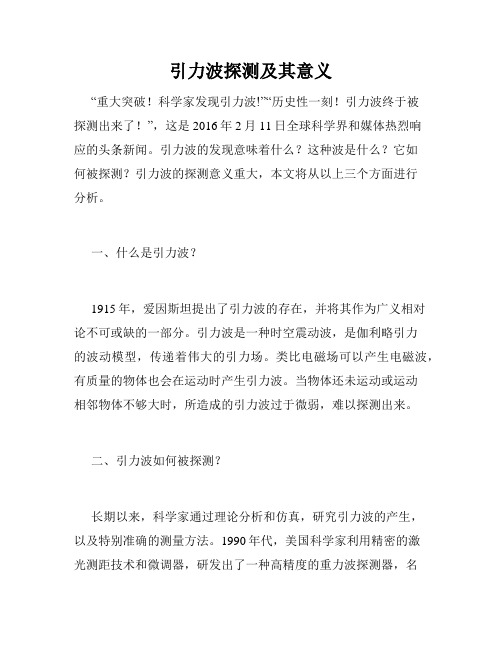
引力波探测及其意义“重大突破!科学家发现引力波!”“历史性一刻!引力波终于被探测出来了!”,这是2016年2月11日全球科学界和媒体热烈响应的头条新闻。
引力波的发现意味着什么?这种波是什么?它如何被探测?引力波的探测意义重大,本文将从以上三个方面进行分析。
一、什么是引力波?1915年,爱因斯坦提出了引力波的存在,并将其作为广义相对论不可或缺的一部分。
引力波是一种时空震动波,是伽利略引力的波动模型,传递着伟大的引力场。
类比电磁场可以产生电磁波,有质量的物体也会在运动时产生引力波。
当物体还未运动或运动相邻物体不够大时,所造成的引力波过于微弱,难以探测出来。
二、引力波如何被探测?长期以来,科学家通过理论分析和仿真,研究引力波的产生,以及特别准确的测量方法。
1990年代,美国科学家利用精密的激光测距技术和微调器,研发出了一种高精度的重力波探测器,名为LIGO(Laser Interferometer Gravitational-Wave Observatory)。
LIGO的核心部分是两条同时垂直的4千米长的高度真空导管,下端各安装有一个高精度激光射频干涉仪;通过两个干涉仪的光程差转换为脉冲信号。
当引力波经过时,它就会扰动基线长度,通过干涉仪接收很弱的光脉冲。
三、引力波探测的意义?引力波探测切实验证了广义相对论中的引力波理论,同时也打开了新的研究领域。
引力波会在行程中被弹撞、反射、扭曲等,所以它们传递的信息可以揭示黑洞和中子星等天体的演化过程,进一步巩固它们的存在和质量、速度等性质。
此外,引力波探测可以帮助解释这个宇宙的起点和结局。
我们知道,最初的大爆炸或许会遗留下引力波痕迹;类似的,随着太阳系内行星的运动,引力波也可能产生调整,使得我们了解更多太阳系演化的细节。
综合来看,引力波探测科学重要性不言而喻。
纵观历史,人类一直在探求宇宙的奥秘,而引力波的探测,为我们揭示了宇宙的一层神秘面纱,人类对于宇宙的了解又得到了一次规模的跨越。
Gravitational waves(引力波)

1 Introduction
2)The violent merging(兼并) of two black holes in deep space
2 Discovery
1)Light interference
2 Discovery
• Video • Beijing time on February 11, 2016 at about 23:40, the laser interferometer gravitational wave observatory (LIGO) responsible person, California Institute of Technology professor announced that the first human discovery of gravitational waves.
Gravitational waves(引力波)
1 Introduction 2 Discovery 3 Application
秦振恒(Life)
1 Introduction
1)Warps(扭曲) space and time
“spacetime”, the way a bowling ball changes the shape of a trampoline(蹦床) as it rolls around on it. Smaller objects will move differently as a result — like marbles spiraling(盘旋) toward a bowlingball-sized dent(凹迹) in a trampoline instead of sitting on a flat surface.
引力波观测原文PhysRevLett.116.061102
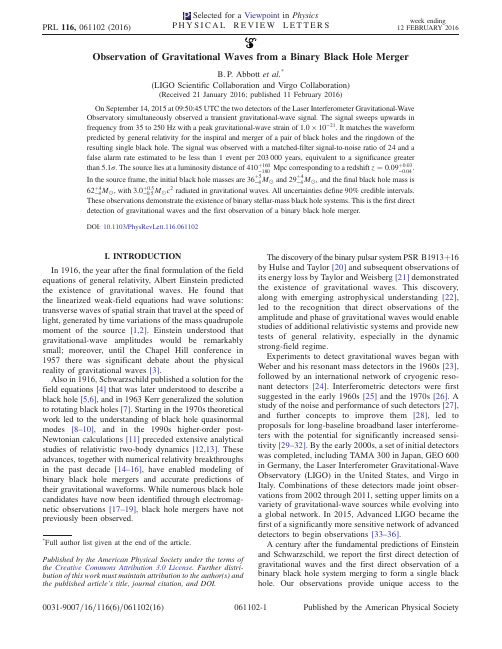
Observation of Gravitational Waves from a Binary Black Hole MergerB.P.Abbott et al.*(LIGO Scientific Collaboration and Virgo Collaboration)(Received21January2016;published11February2016)On September14,2015at09:50:45UTC the two detectors of the Laser Interferometer Gravitational-Wave Observatory simultaneously observed a transient gravitational-wave signal.The signal sweeps upwards in frequency from35to250Hz with a peak gravitational-wave strain of1.0×10−21.It matches the waveform predicted by general relativity for the inspiral and merger of a pair of black holes and the ringdown of the resulting single black hole.The signal was observed with a matched-filter signal-to-noise ratio of24and a false alarm rate estimated to be less than1event per203000years,equivalent to a significance greaterthan5.1σ.The source lies at a luminosity distance of410þ160−180Mpc corresponding to a redshift z¼0.09þ0.03−0.04.In the source frame,the initial black hole masses are36þ5−4M⊙and29þ4−4M⊙,and the final black hole mass is62þ4−4M⊙,with3.0þ0.5−0.5M⊙c2radiated in gravitational waves.All uncertainties define90%credible intervals.These observations demonstrate the existence of binary stellar-mass black hole systems.This is the first direct detection of gravitational waves and the first observation of a binary black hole merger.DOI:10.1103/PhysRevLett.116.061102I.INTRODUCTIONIn1916,the year after the final formulation of the field equations of general relativity,Albert Einstein predicted the existence of gravitational waves.He found that the linearized weak-field equations had wave solutions: transverse waves of spatial strain that travel at the speed of light,generated by time variations of the mass quadrupole moment of the source[1,2].Einstein understood that gravitational-wave amplitudes would be remarkably small;moreover,until the Chapel Hill conference in 1957there was significant debate about the physical reality of gravitational waves[3].Also in1916,Schwarzschild published a solution for the field equations[4]that was later understood to describe a black hole[5,6],and in1963Kerr generalized the solution to rotating black holes[7].Starting in the1970s theoretical work led to the understanding of black hole quasinormal modes[8–10],and in the1990s higher-order post-Newtonian calculations[11]preceded extensive analytical studies of relativistic two-body dynamics[12,13].These advances,together with numerical relativity breakthroughs in the past decade[14–16],have enabled modeling of binary black hole mergers and accurate predictions of their gravitational waveforms.While numerous black hole candidates have now been identified through electromag-netic observations[17–19],black hole mergers have not previously been observed.The discovery of the binary pulsar system PSR B1913þ16 by Hulse and Taylor[20]and subsequent observations of its energy loss by Taylor and Weisberg[21]demonstrated the existence of gravitational waves.This discovery, along with emerging astrophysical understanding[22], led to the recognition that direct observations of the amplitude and phase of gravitational waves would enable studies of additional relativistic systems and provide new tests of general relativity,especially in the dynamic strong-field regime.Experiments to detect gravitational waves began with Weber and his resonant mass detectors in the1960s[23], followed by an international network of cryogenic reso-nant detectors[24].Interferometric detectors were first suggested in the early1960s[25]and the1970s[26].A study of the noise and performance of such detectors[27], and further concepts to improve them[28],led to proposals for long-baseline broadband laser interferome-ters with the potential for significantly increased sensi-tivity[29–32].By the early2000s,a set of initial detectors was completed,including TAMA300in Japan,GEO600 in Germany,the Laser Interferometer Gravitational-Wave Observatory(LIGO)in the United States,and Virgo in binations of these detectors made joint obser-vations from2002through2011,setting upper limits on a variety of gravitational-wave sources while evolving into a global network.In2015,Advanced LIGO became the first of a significantly more sensitive network of advanced detectors to begin observations[33–36].A century after the fundamental predictions of Einstein and Schwarzschild,we report the first direct detection of gravitational waves and the first direct observation of a binary black hole system merging to form a single black hole.Our observations provide unique access to the*Full author list given at the end of the article.Published by the American Physical Society under the terms of the Creative Commons Attribution3.0License.Further distri-bution of this work must maintain attribution to the author(s)and the published article’s title,journal citation,and DOI.properties of space-time in the strong-field,high-velocity regime and confirm predictions of general relativity for the nonlinear dynamics of highly disturbed black holes.II.OBSERVATIONOn September14,2015at09:50:45UTC,the LIGO Hanford,W A,and Livingston,LA,observatories detected the coincident signal GW150914shown in Fig.1.The initial detection was made by low-latency searches for generic gravitational-wave transients[41]and was reported within three minutes of data acquisition[43].Subsequently, matched-filter analyses that use relativistic models of com-pact binary waveforms[44]recovered GW150914as the most significant event from each detector for the observa-tions reported here.Occurring within the10-msintersite FIG.1.The gravitational-wave event GW150914observed by the LIGO Hanford(H1,left column panels)and Livingston(L1,rightcolumn panels)detectors.Times are shown relative to September14,2015at09:50:45UTC.For visualization,all time series are filtered with a35–350Hz bandpass filter to suppress large fluctuations outside the detectors’most sensitive frequency band,and band-reject filters to remove the strong instrumental spectral lines seen in the Fig.3spectra.Top row,left:H1strain.Top row,right:L1strain.GW150914arrived first at L1and6.9þ0.5−0.4ms later at H1;for a visual comparison,the H1data are also shown,shifted in time by this amount and inverted(to account for the detectors’relative orientations).Second row:Gravitational-wave strain projected onto each detector in the35–350Hz band.Solid lines show a numerical relativity waveform for a system with parameters consistent with those recovered from GW150914[37,38]confirmed to99.9%by an independent calculation based on[15].Shaded areas show90%credible regions for two independent waveform reconstructions.One(dark gray)models the signal using binary black hole template waveforms [39].The other(light gray)does not use an astrophysical model,but instead calculates the strain signal as a linear combination of sine-Gaussian wavelets[40,41].These reconstructions have a94%overlap,as shown in[39].Third row:Residuals after subtracting the filtered numerical relativity waveform from the filtered detector time series.Bottom row:A time-frequency representation[42]of the strain data,showing the signal frequency increasing over time.propagation time,the events have a combined signal-to-noise ratio(SNR)of24[45].Only the LIGO detectors were observing at the time of GW150914.The Virgo detector was being upgraded, and GEO600,though not sufficiently sensitive to detect this event,was operating but not in observational mode.With only two detectors the source position is primarily determined by the relative arrival time and localized to an area of approximately600deg2(90% credible region)[39,46].The basic features of GW150914point to it being produced by the coalescence of two black holes—i.e., their orbital inspiral and merger,and subsequent final black hole ringdown.Over0.2s,the signal increases in frequency and amplitude in about8cycles from35to150Hz,where the amplitude reaches a maximum.The most plausible explanation for this evolution is the inspiral of two orbiting masses,m1and m2,due to gravitational-wave emission.At the lower frequencies,such evolution is characterized by the chirp mass[11]M¼ðm1m2Þ3=5121=5¼c3G596π−8=3f−11=3_f3=5;where f and_f are the observed frequency and its time derivative and G and c are the gravitational constant and speed of light.Estimating f and_f from the data in Fig.1, we obtain a chirp mass of M≃30M⊙,implying that the total mass M¼m1þm2is≳70M⊙in the detector frame. This bounds the sum of the Schwarzschild radii of thebinary components to2GM=c2≳210km.To reach an orbital frequency of75Hz(half the gravitational-wave frequency)the objects must have been very close and very compact;equal Newtonian point masses orbiting at this frequency would be only≃350km apart.A pair of neutron stars,while compact,would not have the required mass,while a black hole neutron star binary with the deduced chirp mass would have a very large total mass, and would thus merge at much lower frequency.This leaves black holes as the only known objects compact enough to reach an orbital frequency of75Hz without contact.Furthermore,the decay of the waveform after it peaks is consistent with the damped oscillations of a black hole relaxing to a final stationary Kerr configuration. Below,we present a general-relativistic analysis of GW150914;Fig.2shows the calculated waveform using the resulting source parameters.III.DETECTORSGravitational-wave astronomy exploits multiple,widely separated detectors to distinguish gravitational waves from local instrumental and environmental noise,to provide source sky localization,and to measure wave polarizations. The LIGO sites each operate a single Advanced LIGO detector[33],a modified Michelson interferometer(see Fig.3)that measures gravitational-wave strain as a differ-ence in length of its orthogonal arms.Each arm is formed by two mirrors,acting as test masses,separated by L x¼L y¼L¼4km.A passing gravitational wave effec-tively alters the arm lengths such that the measured difference isΔLðtÞ¼δL x−δL y¼hðtÞL,where h is the gravitational-wave strain amplitude projected onto the detector.This differential length variation alters the phase difference between the two light fields returning to the beam splitter,transmitting an optical signal proportional to the gravitational-wave strain to the output photodetector. To achieve sufficient sensitivity to measure gravitational waves,the detectors include several enhancements to the basic Michelson interferometer.First,each arm contains a resonant optical cavity,formed by its two test mass mirrors, that multiplies the effect of a gravitational wave on the light phase by a factor of300[48].Second,a partially trans-missive power-recycling mirror at the input provides addi-tional resonant buildup of the laser light in the interferometer as a whole[49,50]:20W of laser input is increased to700W incident on the beam splitter,which is further increased to 100kW circulating in each arm cavity.Third,a partially transmissive signal-recycling mirror at the outputoptimizes FIG. 2.Top:Estimated gravitational-wave strain amplitude from GW150914projected onto H1.This shows the full bandwidth of the waveforms,without the filtering used for Fig.1. The inset images show numerical relativity models of the black hole horizons as the black holes coalesce.Bottom:The Keplerian effective black hole separation in units of Schwarzschild radii (R S¼2GM=c2)and the effective relative velocity given by the post-Newtonian parameter v=c¼ðGMπf=c3Þ1=3,where f is the gravitational-wave frequency calculated with numerical relativity and M is the total mass(value from Table I).the gravitational-wave signal extraction by broadening the bandwidth of the arm cavities [51,52].The interferometer is illuminated with a 1064-nm wavelength Nd:Y AG laser,stabilized in amplitude,frequency,and beam geometry [53,54].The gravitational-wave signal is extracted at the output port using a homodyne readout [55].These interferometry techniques are designed to maxi-mize the conversion of strain to optical signal,thereby minimizing the impact of photon shot noise (the principal noise at high frequencies).High strain sensitivity also requires that the test masses have low displacement noise,which is achieved by isolating them from seismic noise (low frequencies)and designing them to have low thermal noise (intermediate frequencies).Each test mass is suspended as the final stage of a quadruple-pendulum system [56],supported by an active seismic isolation platform [57].These systems collectively provide more than 10orders of magnitude of isolation from ground motion for frequen-cies above 10Hz.Thermal noise is minimized by using low-mechanical-loss materials in the test masses and their suspensions:the test masses are 40-kg fused silica substrates with low-loss dielectric optical coatings [58,59],and are suspended with fused silica fibers from the stage above [60].To minimize additional noise sources,all components other than the laser source are mounted on vibration isolation stages in ultrahigh vacuum.To reduce optical phase fluctuations caused by Rayleigh scattering,the pressure in the 1.2-m diameter tubes containing the arm-cavity beams is maintained below 1μPa.Servo controls are used to hold the arm cavities on resonance [61]and maintain proper alignment of the optical components [62].The detector output is calibrated in strain by measuring its response to test mass motion induced by photon pressure from a modulated calibration laser beam [63].The calibration is established to an uncertainty (1σ)of less than 10%in amplitude and 10degrees in phase,and is continuously monitored with calibration laser excitations at selected frequencies.Two alternative methods are used to validate the absolute calibration,one referenced to the main laser wavelength and the other to a radio-frequencyoscillator(a)FIG.3.Simplified diagram of an Advanced LIGO detector (not to scale).A gravitational wave propagating orthogonally to the detector plane and linearly polarized parallel to the 4-km optical cavities will have the effect of lengthening one 4-km arm and shortening the other during one half-cycle of the wave;these length changes are reversed during the other half-cycle.The output photodetector records these differential cavity length variations.While a detector ’s directional response is maximal for this case,it is still significant for most other angles of incidence or polarizations (gravitational waves propagate freely through the Earth).Inset (a):Location and orientation of the LIGO detectors at Hanford,WA (H1)and Livingston,LA (L1).Inset (b):The instrument noise for each detector near the time of the signal detection;this is an amplitude spectral density,expressed in terms of equivalent gravitational-wave strain amplitude.The sensitivity is limited by photon shot noise at frequencies above 150Hz,and by a superposition of other noise sources at lower frequencies [47].Narrow-band features include calibration lines (33–38,330,and 1080Hz),vibrational modes of suspension fibers (500Hz and harmonics),and 60Hz electric power grid harmonics.[64].Additionally,the detector response to gravitational waves is tested by injecting simulated waveforms with the calibration laser.To monitor environmental disturbances and their influ-ence on the detectors,each observatory site is equipped with an array of sensors:seismometers,accelerometers, microphones,magnetometers,radio receivers,weather sensors,ac-power line monitors,and a cosmic-ray detector [65].Another∼105channels record the interferometer’s operating point and the state of the control systems.Data collection is synchronized to Global Positioning System (GPS)time to better than10μs[66].Timing accuracy is verified with an atomic clock and a secondary GPS receiver at each observatory site.In their most sensitive band,100–300Hz,the current LIGO detectors are3to5times more sensitive to strain than initial LIGO[67];at lower frequencies,the improvement is even greater,with more than ten times better sensitivity below60Hz.Because the detectors respond proportionally to gravitational-wave amplitude,at low redshift the volume of space to which they are sensitive increases as the cube of strain sensitivity.For binary black holes with masses similar to GW150914,the space-time volume surveyed by the observations reported here surpasses previous obser-vations by an order of magnitude[68].IV.DETECTOR VALIDATIONBoth detectors were in steady state operation for several hours around GW150914.All performance measures,in particular their average sensitivity and transient noise behavior,were typical of the full analysis period[69,70]. Exhaustive investigations of instrumental and environ-mental disturbances were performed,giving no evidence to suggest that GW150914could be an instrumental artifact [69].The detectors’susceptibility to environmental disturb-ances was quantified by measuring their response to spe-cially generated magnetic,radio-frequency,acoustic,and vibration excitations.These tests indicated that any external disturbance large enough to have caused the observed signal would have been clearly recorded by the array of environ-mental sensors.None of the environmental sensors recorded any disturbances that evolved in time and frequency like GW150914,and all environmental fluctuations during the second that contained GW150914were too small to account for more than6%of its strain amplitude.Special care was taken to search for long-range correlated disturbances that might produce nearly simultaneous signals at the two sites. No significant disturbances were found.The detector strain data exhibit non-Gaussian noise transients that arise from a variety of instrumental mecha-nisms.Many have distinct signatures,visible in auxiliary data channels that are not sensitive to gravitational waves; such instrumental transients are removed from our analyses [69].Any instrumental transients that remain in the data are accounted for in the estimated detector backgrounds described below.There is no evidence for instrumental transients that are temporally correlated between the two detectors.V.SEARCHESWe present the analysis of16days of coincident observations between the two LIGO detectors from September12to October20,2015.This is a subset of the data from Advanced LIGO’s first observational period that ended on January12,2016.GW150914is confidently detected by two different types of searches.One aims to recover signals from the coalescence of compact objects,using optimal matched filtering with waveforms predicted by general relativity. The other search targets a broad range of generic transient signals,with minimal assumptions about waveforms.These searches use independent methods,and their response to detector noise consists of different,uncorrelated,events. However,strong signals from binary black hole mergers are expected to be detected by both searches.Each search identifies candidate events that are detected at both observatories consistent with the intersite propa-gation time.Events are assigned a detection-statistic value that ranks their likelihood of being a gravitational-wave signal.The significance of a candidate event is determined by the search background—the rate at which detector noise produces events with a detection-statistic value equal to or higher than the candidate event.Estimating this back-ground is challenging for two reasons:the detector noise is nonstationary and non-Gaussian,so its properties must be empirically determined;and it is not possible to shield the detector from gravitational waves to directly measure a signal-free background.The specific procedure used to estimate the background is slightly different for the two searches,but both use a time-shift technique:the time stamps of one detector’s data are artificially shifted by an offset that is large compared to the intersite propagation time,and a new set of events is produced based on this time-shifted data set.For instrumental noise that is uncor-related between detectors this is an effective way to estimate the background.In this process a gravitational-wave signal in one detector may coincide with time-shifted noise transients in the other detector,thereby contributing to the background estimate.This leads to an overestimate of the noise background and therefore to a more conservative assessment of the significance of candidate events.The characteristics of non-Gaussian noise vary between different time-frequency regions.This means that the search backgrounds are not uniform across the space of signals being searched.To maximize sensitivity and provide a better estimate of event significance,the searches sort both their background estimates and their event candidates into differ-ent classes according to their time-frequency morphology. The significance of a candidate event is measured against the background of its class.To account for having searchedmultiple classes,this significance is decreased by a trials factor equal to the number of classes [71].A.Generic transient searchDesigned to operate without a specific waveform model,this search identifies coincident excess power in time-frequency representations of the detector strain data [43,72],for signal frequencies up to 1kHz and durations up to a few seconds.The search reconstructs signal waveforms consistent with a common gravitational-wave signal in both detectors using a multidetector maximum likelihood method.Each event is ranked according to the detection statistic ηc ¼ffiffiffiffiffiffiffiffiffiffiffiffiffiffiffiffiffiffiffiffiffiffiffiffiffiffiffiffiffiffiffiffiffiffiffi2E c =ð1þE n =E c Þp ,where E c is the dimensionless coherent signal energy obtained by cross-correlating the two reconstructed waveforms,and E n is the dimensionless residual noise energy after the reconstructed signal is subtracted from the data.The statistic ηc thus quantifies the SNR of the event and the consistency of the data between the two detectors.Based on their time-frequency morphology,the events are divided into three mutually exclusive search classes,as described in [41]:events with time-frequency morphology of known populations of noise transients (class C1),events with frequency that increases with time (class C3),and all remaining events (class C2).Detected with ηc ¼20.0,GW150914is the strongest event of the entire search.Consistent with its coalescence signal signature,it is found in the search class C3of events with increasing time-frequency evolution.Measured on a background equivalent to over 67400years of data and including a trials factor of 3to account for the search classes,its false alarm rate is lower than 1in 22500years.This corresponds to a probability <2×10−6of observing one or more noise events as strong as GW150914during the analysis time,equivalent to 4.6σ.The left panel of Fig.4shows the C3class results and background.The selection criteria that define the search class C3reduce the background by introducing a constraint on the signal morphology.In order to illustrate the significance of GW150914against a background of events with arbitrary shapes,we also show the results of a search that uses the same set of events as the one described above but without this constraint.Specifically,we use only two search classes:the C1class and the union of C2and C3classes (C 2þC 3).In this two-class search the GW150914event is found in the C 2þC 3class.The left panel of Fig.4shows the C 2þC 3class results and background.In the background of this class there are four events with ηc ≥32.1,yielding a false alarm rate for GW150914of 1in 8400years.This corresponds to a false alarm probability of 5×10−6equivalent to 4.4σ.FIG.4.Search results from the generic transient search (left)and the binary coalescence search (right).These histograms show the number of candidate events (orange markers)and the mean number of background events (black lines)in the search class where GW150914was found as a function of the search detection statistic and with a bin width of 0.2.The scales on the top give the significance of an event in Gaussian standard deviations based on the corresponding noise background.The significance of GW150914is greater than 5.1σand 4.6σfor the binary coalescence and the generic transient searches,respectively.Left:Along with the primary search (C3)we also show the results (blue markers)and background (green curve)for an alternative search that treats events independently of their frequency evolution (C 2þC 3).The classes C2and C3are defined in the text.Right:The tail in the black-line background of the binary coalescence search is due to random coincidences of GW150914in one detector with noise in the other detector.(This type of event is practically absent in the generic transient search background because they do not pass the time-frequency consistency requirements used in that search.)The purple curve is the background excluding those coincidences,which is used to assess the significance of the second strongest event.For robustness and validation,we also use other generic transient search algorithms[41].A different search[73]and a parameter estimation follow-up[74]detected GW150914 with consistent significance and signal parameters.B.Binary coalescence searchThis search targets gravitational-wave emission from binary systems with individual masses from1to99M⊙, total mass less than100M⊙,and dimensionless spins up to 0.99[44].To model systems with total mass larger than 4M⊙,we use the effective-one-body formalism[75],whichcombines results from the post-Newtonian approach [11,76]with results from black hole perturbation theory and numerical relativity.The waveform model[77,78] assumes that the spins of the merging objects are alignedwith the orbital angular momentum,but the resultingtemplates can,nonetheless,effectively recover systemswith misaligned spins in the parameter region ofGW150914[44].Approximately250000template wave-forms are used to cover this parameter space.The search calculates the matched-filter signal-to-noiseratioρðtÞfor each template in each detector and identifiesmaxima ofρðtÞwith respect to the time of arrival of the signal[79–81].For each maximum we calculate a chi-squared statisticχ2r to test whether the data in several differentfrequency bands are consistent with the matching template [82].Values ofχ2r near unity indicate that the signal is consistent with a coalescence.Ifχ2r is greater than unity,ρðtÞis reweighted asˆρ¼ρ=f½1þðχ2rÞ3 =2g1=6[83,84].The final step enforces coincidence between detectors by selectingevent pairs that occur within a15-ms window and come fromthe same template.The15-ms window is determined by the10-ms intersite propagation time plus5ms for uncertainty inarrival time of weak signals.We rank coincident events basedon the quadrature sumˆρc of theˆρfrom both detectors[45]. To produce background data for this search the SNR maxima of one detector are time shifted and a new set of coincident events is computed.Repeating this procedure ∼107times produces a noise background analysis time equivalent to608000years.To account for the search background noise varying acrossthe target signal space,candidate and background events aredivided into three search classes based on template length.The right panel of Fig.4shows the background for thesearch class of GW150914.The GW150914detection-statistic value ofˆρc¼23.6is larger than any background event,so only an upper bound can be placed on its false alarm rate.Across the three search classes this bound is1in 203000years.This translates to a false alarm probability <2×10−7,corresponding to5.1σ.A second,independent matched-filter analysis that uses adifferent method for estimating the significance of itsevents[85,86],also detected GW150914with identicalsignal parameters and consistent significance.When an event is confidently identified as a real gravitational-wave signal,as for GW150914,the back-ground used to determine the significance of other events is reestimated without the contribution of this event.This is the background distribution shown as a purple line in the right panel of Fig.4.Based on this,the second most significant event has a false alarm rate of1per2.3years and corresponding Poissonian false alarm probability of0.02. Waveform analysis of this event indicates that if it is astrophysical in origin it is also a binary black hole merger[44].VI.SOURCE DISCUSSIONThe matched-filter search is optimized for detecting signals,but it provides only approximate estimates of the source parameters.To refine them we use general relativity-based models[77,78,87,88],some of which include spin precession,and for each model perform a coherent Bayesian analysis to derive posterior distributions of the source parameters[89].The initial and final masses, final spin,distance,and redshift of the source are shown in Table I.The spin of the primary black hole is constrained to be<0.7(90%credible interval)indicating it is not maximally spinning,while the spin of the secondary is only weakly constrained.These source parameters are discussed in detail in[39].The parameter uncertainties include statistical errors and systematic errors from averaging the results of different waveform models.Using the fits to numerical simulations of binary black hole mergers in[92,93],we provide estimates of the mass and spin of the final black hole,the total energy radiated in gravitational waves,and the peak gravitational-wave luminosity[39].The estimated total energy radiated in gravitational waves is3.0þ0.5−0.5M⊙c2.The system reached apeak gravitational-wave luminosity of3.6þ0.5−0.4×1056erg=s,equivalent to200þ30−20M⊙c2=s.Several analyses have been performed to determine whether or not GW150914is consistent with a binary TABLE I.Source parameters for GW150914.We report median values with90%credible intervals that include statistical errors,and systematic errors from averaging the results of different waveform models.Masses are given in the source frame;to convert to the detector frame multiply by(1þz) [90].The source redshift assumes standard cosmology[91]. Primary black hole mass36þ5−4M⊙Secondary black hole mass29þ4−4M⊙Final black hole mass62þ4−4M⊙Final black hole spin0.67þ0.05−0.07 Luminosity distance410þ160−180MpcSource redshift z0.09þ0.03−0.04。
2022-2023学年江西省顶级名校高三下学期一模考试英语试题含解析

2023年高考英语模拟试卷注意事项:1.答卷前,考生务必将自己的姓名、准考证号填写在答题卡上。
2.回答选择题时,选出每小题答案后,用铅笔把答题卡上对应题目的答案标号涂黑,如需改动,用橡皮擦干净后,再选涂其它答案标号。
回答非选择题时,将答案写在答题卡上,写在本试卷上无效。
3.考试结束后,将本试卷和答题卡一并交回。
第一部分(共20小题,每小题1.5分,满分30分)1.I hope my teacher will take into _______ the fact that I was ill just before the exams when she marks my paper. A.idea B.considered C.account D.thought2.语音知识(共5小题;每小题l分,满分5分)从A、B、C、D四个选项中,找出其划线部分与所给单词的划线部分读音相同的选项。
并在答题卡上将该项涂黑。
3.Pandas are _____ to the mountains of central China and only about 1,000 remain in the wild.A. native B.sensitive C.relate D.familiar4.So absorbed ________ in her yoga exercises that she took no notice of the heavy rain outside.A.Mary was B.Mary has beenC.was Mary D.has Mary been5.______ property, we’re among the richest people in this city.A.In search of B.In spite of C.In place of D.In terms of6.We are to hold the sports meeting next weekend, ________ the air quality becomes better.A.which B.whenC.where D.while7.It is one thing to enjoy listening to good music, but it is quite ______ to perform skillfully yourself.A.another B.other C.the other D.others8.—What a shame! We misunderstood each other for such a long time.—Yes, I wish I _____ with you earlier.A.communicate B.had communicatedC.communicated D.would communicate9.During each NBA season, basketball fans cheer on their favorite teams to make _______ through.A.it B.themC.that D.those10.People tend to love agricultural products ________ without the use of fertilizers, pesticides or chemical additives. A.growing B.grownC.being grown D.having been grown11.It rained this morning, _____ actually didn’t bother me because I like walking in the rain.A.what B.whenC.where D.which12.The first snow didn’t fall until February in our province this year, ________ was unexpec ted.A.it B.which C.that D.what13.The disaster-stricken village was inaccessible ___________ by helicopter, and the storm added to the rescuers’ difficulty.A.instead of B.other than C.rather than D.regardless of14.—Did you enjoy your journey to Beijing last weekend?—. We had driven more than 3 hours before we found the right way.A.Absolutely B.No way C.Not at all D.With pleasure15.Regarding China-US differences on human rights issues, Hong said the two sides can enhance mutual understanding through dialogue ______ on equality and mutual respect.A.based B.to base C.basing D.base16.—Do you know when your mother ________ to pick you up?—At 11:40 am.A.had come B.is comingC.has come D.would come17.—Hi, Tom! I got a chance to be an exchange student in Harvard University.—_________! I had been expecting to study there.A.Lucky you B.Have funC.Take it easy D.Forget it18.The government officials met the workers and engineers working on the stadium, most____ were migrant workers.A.of which B.of who C.of whom D.of them19.—I’m sorry for breaking the cup.—Oh, ________. I’ve got plenty.A.help yourself B.forget itC.my pleasure D.pardon me20.—Got your driving license?—No. I too busy to have enough practice, so I didn’t take the driving test last week.A.was B.amC.have been D.had been第二部分阅读理解(满分40分)阅读下列短文,从每题所给的A、B、C、D四个选项中,选出最佳选项。
引力波的探索与发现:2024年科学突破总结

引力波的探索与发现:2024年科学突破总结Introduction:1. Overview:Gravity waves, a concept that fascinated scientists for decades, have finally been observed and confirmed in recent years. This breakthrough has opened up new avenues for exploring the mysteries of the universe. In this article, we will summarize the exploration and discovery of gravity waves up until 2024.2. Research Background:Gravity waves were first predicted by Albert Einstein in his General Theory of Relativity over a century ago. According to Einstein's theory, these waves are ripples in the fabric of spacetime caused by massive objects accelerating. Despite this theoretical prediction, it took several decades to develop the technology required to detect and study gravity waves.3. Purpose and Significance:The purpose of this article is to provide an overview of the journey towards the discovery of gravity waves and highlight its scientificsignificance. By understanding the process and technological advancements involved in detecting these waves, we can appreciate the profound impact they have had on our understanding of astrophysics and the origins of the universe.Kindly note that "..." indicates where you can add more specific information or expand on certain points based on your research about gravity wave exploration and discovery until 2024.2. 引力波的发现历程2.1 爱因斯坦的预言引力波是由爱因斯坦在他的广义相对论理论中预言的一种激动传播物质与能量引起的时空弯曲效应。
江苏省七校联盟2024学年高三考前热身英语试卷(含解析)
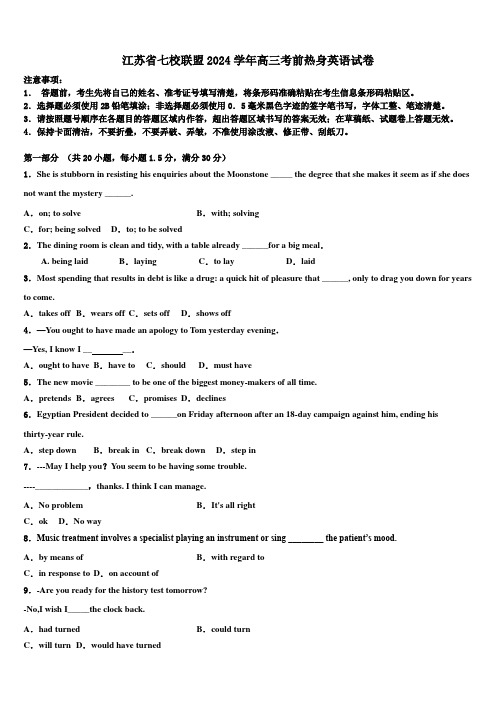
江苏省七校联盟2024学年高三考前热身英语试卷注意事项:1.答题前,考生先将自己的姓名、准考证号填写清楚,将条形码准确粘贴在考生信息条形码粘贴区。
2.选择题必须使用2B铅笔填涂;非选择题必须使用0.5毫米黑色字迹的签字笔书写,字体工整、笔迹清楚。
3.请按照题号顺序在各题目的答题区域内作答,超出答题区域书写的答案无效;在草稿纸、试题卷上答题无效。
4.保持卡面清洁,不要折叠,不要弄破、弄皱,不准使用涂改液、修正带、刮纸刀。
第一部分(共20小题,每小题1.5分,满分30分)1.She is stubborn in resisting his enquiries about the Moonstone _____ the degree that she makes it seem as if she does not want the mystery ______.A.on; to solve B.with; solvingC.for; being solved D.to; to be solved2.The dining room is clean and tidy, with a table already ______for a big meal.A. being laid B.laying C.to lay D.laid3.Most spending that results in debt is like a drug: a quick hit of pleasure that ______, only to drag you down for years to come.A.takes off B.wears off C.sets off D.shows off4.—You ought to have made an apology to Tom yesterday evening.—Yes, I know I __ __.A.ought to have B.have to C.should D.must have5.The new movie ________ to be one of the biggest money-makers of all time.A.pretends B.agrees C.promises D.declines6.Egyptian President decided to ______on Friday afternoon after an 18-day campaign against him, ending histhirty-year rule.A.step down B.break in C.break down D.step in7.---May I help you?You seem to be having some trouble.----____________,thanks. I think I can manage.A.No problem B.It's all rightC.ok D.No way8.Music treatment involves a specialist playing an instrument or sing ________ the patient’s mood.A.by means of B.with regard toC.in response to D.on account of9.-Are you ready for the history test tomorrow?-No,I wish I_____the clock back.A.had turned B.could turnC.will turn D.would have turned10.They felt ________ it was high tax and low income ________ contributed to the extreme misery of the working people at the bottom of the ladder.A./;that B.that; whichC.that; what D./; which11.China’s BeiDou Navigation Satellite System, whose positioning ________ will reach 2.5 meters by 2020, will soon provide services for more countries.A.accuracy B.categoryC.function D.reference12.She was so angry and spoke so fast that none of us understood ______ he said meant.A.that B.what C.that that D.what what13.I’m interested in a blue dress. Do you have any _______?A.convenient B.available C.possible D.personal14.According to the co mpany’s rule, one’s payment is ______ the work done, not to the time spent doing it.A.in proportion to B.in addition toC.in contrast to D.in regard to15.This raw chocolate tastes pretty delicious due to ______ amount of melted pure fresh cream.A.equal B.generous C.insufficient D.tiny16.The 2011 Australian Open was successfully held in city of Melbourne, big city in Australia.A.a; a B.the; a C.a; the D.the; the17.Never turn down a job because you think it’s too small. You don’t know _____ it can lead.A.how B.whereC.whether D.what18.The customs officers were insisting that suitcases should be opened and their contents _______ for closer inspection. A.laid out B.given out C.sent out D.picked out19.— What great changes have taken place in our city in the last few years!— Indeed, many high buildings have _______all over the city.A.wound up B.sprung up C.held up D.made up20.We ______ be careful with the words we say when we are angry.A.may B.can C.might D.should第二部分阅读理解(满分40分)阅读下列短文,从每题所给的A、B、C、D四个选项中,选出最佳选项。
新标准大学英语视听说教程(2)听力原文_Unit+6+New
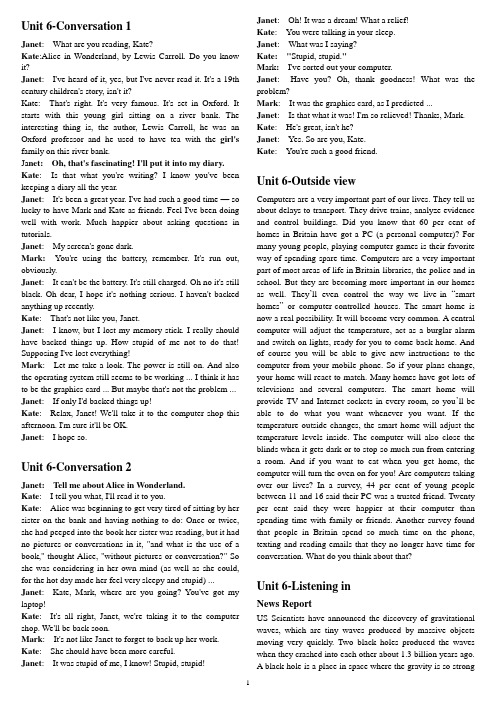
Unit 6-Conversation 1Janet: What are you reading, Kate?Kate:Alice in Wonderland, by Lewis Carroll. Do you know it?Janet: I've heard of it, yes, but I've never read it. It's a 19th century children's story, isn't it?K a te: That's right. It's very famous. It's set in Oxford. It starts with this young girl sitting on a river bank. The interesting thing is, the author, Lewis Carroll, he was an Oxford professor and he used to have tea with the girl's family on this river bank.Ja net: Oh, that's fascinating! I'll put it into my diary. Kate: Is that what you're writing? I know you've been keeping a diary all the year.Janet: It's been a great year. I've had such a good time — so lucky to have Mark and Kate as friends. Feel I've been doing well with work. Much happier about asking questions in tutorials.Janet: My screen's gone dark.Mark: You're using the battery, remember. It's run out, obviously.Janet: It can't be the battery. It's still charged. Oh no it's still black. Oh dear, I hope it's nothing serious. I haven't backed anything up recently.Kate: That's not like you, Janet.Janet:I know, but I lost my memory stick. I really should have backed things up. How stupid of me not to do that! Supposing I've lost everything!Mark: Let me take a look. The power is still on. And also the operating system still seems to be working ... I think it has to be the graphics card ... But maybe that's not the problem ... Janet: If only I'd backed things up!Kate: Relax, Janet! We'll take it to the computer shop this afternoon. I'm sure it'll be OK.Janet: I hope so.Unit 6-Conversation 2Janet: Tell me about Alice in Wonderland.Kate: I tell you what, I'll read it to you.Kate: Alice was beginning to get very tired of sitting by her sister on the bank and having nothing to do: Once or twice, she had peeped into the book her sister was reading, but it had no pictures or conversations in it, "and what is the use of a book," thought Alice, "without pictures or conversation?" So she was considering in her own mind (as well as she could, for the hot day made her feel very sleepy and stupid) ... Janet: Kate, Mark, where are you going? You've got my laptop!Kate: It's all right, Janet, we're taking it to the computer shop. We'll be back soon.Mark: It's not like Janet to forget to back up her work. Kate: She should have been more careful.Janet: It was stupid of me, I know! Stupid, stupid!Janet: Oh! It was a dream! What a relief!Kate: You were talking in your sleep.Janet: What was I saying?Kate: "Stupid, stupid."M ark:I've sorted out your computer.Janet: Have you? Oh, thank goodness! What was the problem?Mark:It was the graphics card, as I predicted ...Janet: Is that what it was! I'm so relieved! Thanks, Mark.Kate: He's great, isn't he?Janet: Yes. So are you, Kate.Kate: You're such a good friend.Unit 6-Outside viewComputers are a very important part of our lives. They tell us about delays to transport. They drive trains, analyze evidence and control buildings. Did you know that 60 per cent of homes in Britain have got a PC (a personal computer)? For many young people, playing computer games is their favorite way of spending spare time. Computers are a very important part of most areas of life in Britain-libraries, the police and in school. But they are becoming more important in our homes as well. They’ll even control the way we live-in “smart homes”or computer-controlled houses. The smart home is now a real possibility. It will become very common. A central computer will adjust the temperature, act as a burglar alarm and switch on lights, ready for you to come back home. And of course you will be able to give new instructions to the computer from your mobile phone. So if your plans change, your home will react to match. Many homes have got lots of televisions and several computers. The smart home will provide TV and Internet sockets in every room, so you’ll be able to do what you want whenever you want. If the temperature outside changes, the smart home will adjust the temperature levels inside. The computer will also close the blinds when it gets dark or to stop so much sun from enteringa room. And if you want to eat when you get home, thecomputer will turn the oven on for you! Are computers taking over our lives? In a survey, 44 per cent of young people between 11 and 16 said their PC was a trusted friend. Twenty per cent said they were happier at their computer than spending time with family or friends. Another survey found that people in Britain spend so much time on the phone, texting and reading emails that they no longer have time for conversation. What do you think about that?Unit 6-Listening inNews ReportUS Scientists have announced the discovery of gravitational waves, which are tiny waves produced by massive objects moving very quickly. Two black holes produced the waves when they crashed into each other about 1.3 billion years ago.A black hole is a place in space where the gravity is so strong1that even light cannot escape. This announcement of the discovery comes a century after Albert Einstein first predicted gravitational waves would exist.The discovery was made possible by using a highly sensitive instrument designed to detect signals of gravitational waves and identify their sources. This discovery proves that there are gravitational waves, and strongly confirms the existence of black holes.With this discovery, scientists are given a new tool to study and understand the universe. The waves could help scientists learn more about what happened immediately after the universe began and how the universe expanded. Scientists hope that they may be able to observe parts of the universe that were previously undetectable.1.What discovery have US scientists made?2.What features do black holes have according to the newsreport?3.Why is the discovery important?Passage 1When you have a biscuit with your cup of tea, do you dunk it? And if so, what’s the perfect way to do it? That’s the subject of today’s Science in Action report. It may be hard to believe but scientists at the University of Bristol have been analyzing this question. And after a two-month study they devised a mathematical formula for dunking biscuits. So no more melting chocolate, or biscuit crumbs in the bottom of your cup, which is the fate of one in four biscuits that are dunked in tea, according to research by a biscuit manufacturer. Doughnut dunkers don’t face the same problems because doughnuts are held together with an elastic net of protein, gluten. This substance allows the doughnut to absorb liquid without breaking down its structure. The structure of a biscuit, however, is held together by sugar which melts when placed in hot tea or coffee.So what is the answer? The researcher, let by Dr. Len Fisher, discovered that holding the biscuit in a horizontal position – or “flat-on”– has a significant effect on the amount of time that a biscuit can stay in hot liquid before falling apart. In fact this horizontal dunking results in a dunking time up to four times longer than traditional vertical dunking.What’s the reason for this? It seems that the answer is related to diffusion, in other words, the length of time it takes for the liquid to penetrate the structure of the biscuit. Basically, it takes longer for the liquid to travel through the channels of a biscuit when it is laid flat on the surface of the liquid. Also the fact that when a biscuit is dunked horizontally, with the biscuit submerged in the liquid, and the chocolate coating staying out of the liquid, the chocolate helps hold the biscuit together. Another factor influencing the equation is the temperature of the tea – the hotter the tea, the faster the sugar melts.Researchers also found that by dunking a biscuit into tea or coffee, up to ten times more flavor is release than it the biscuit is eaten dry. So it’s worth experimenting yourself. If you are wondering how you can perfect the horizontal dunk, the researchers have come up with an idea for a biscuit-holding device to make dunking biscuits easier. They are even mow working on producing a table giving guidelines on dunking times for different types of biscuit. On that note, I think it’s time to go off to the canteen for a tea break! Passage 2Peter: Hey Louise, look at this book about crop circles - some of the photos are absolutely unbelievable.Louise: You don t believe in all that stuff, do you Peter?Peter: I'm not saying I believe in UFOs and things, but some of the formations are fascinating. They’remade up of lots of interconnected circles andgeometrical shapes. You know, in the past few years,there have been more reports of them. The circlesare getting larger and the designs are getting moreintricate... I'm sure that they can't all be man-made.Think about it - they're so complicated, and theyappear at night in the middle of fields of wheatbarley or corn. It’s definitely pretty weird!Louise: I know, but l saw a TV documentary about it, and they showed how a group of hoaxers made anelaborate crop circle in a field at night usingwooden plank, ropes, plastic tubes and a gardenroller. They even fooled some of the people whobelieve in the paranormal-aliens coming down inUFOs and aliens coming down in UFOs andcreating them, and so on.Peter: I'm sure lots of them are created by people just to get publicity but look here-it says, “The firstrecords of crop circles go back as far as the 17thcentury. Since the 1970s there have been over12,000 reports from countries all around the worldincluding Italy, America, South Africa, Australiaand Brazil.” Most reports are from here in Englandthough.Louise: But surely that’s just because they get so muchmedia coverage these days, so more people aremaking them.Peter: Perhaps, but how do you explain the fact that theactual chemical composition of the grains of corpsinside the circles changes? Scientific tests havefound they have a higher protein level. The stems ofthe grains have often been exposed to hightemperatures. And they found that the soil withinthe circles contains more iron than the soil outside.So far, the hoaxers haven't been able to copy allthese features.Louise: Well, I'm not a scientist but I'm pretty sceptical about all these so-called paranormal explanations. Iremember in the programme I watched, theresearchers found signs of human interference, such2as holes in the earth and footprints!Peter: Come on… you must admit, that still leaves a lot which is unexplained!Louise: There's lots of things that are hard to explain but this really...3。
引力波(gravitational waves)
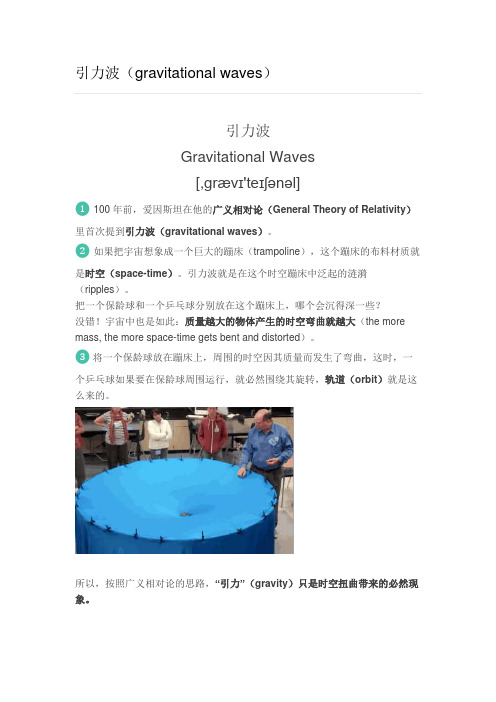
引力波Gravitational Waves[,ɡrævɪ'teɪʃənəl]❶100年前,爱因斯坦在他的广义相对论(General Theory of Relativity)里首次提到引力波(gravitational waves)。
❷如果把宇宙想象成一个巨大的蹦床(trampoline),这个蹦床的布料材质就是时空(space-time)。
引力波就是在这个时空蹦床中泛起的涟漪(ripples)。
把一个保龄球和一个乒乓球分别放在这个蹦床上,哪个会沉得深一些?没错!宇宙中也是如此:质量越大的物体产生的时空弯曲就越大(the more mass, the more space-time gets bent and distorted)。
❸将一个保龄球放在蹦床上,周围的时空因其质量而发生了弯曲,这时,一个乒乓球如果要在保龄球周围运行,就必然围绕其旋转,轨道(orbit)就是这么来的。
所以,按照广义相对论的思路,“引力”(gravity)只是时空扭曲带来的必然现象。
❹当两个巨大物质朝着彼此高速旋转时(spiral toward each other),它们就会以光速在周围扭曲的时空中传播出一波一波的涟漪(they send waves along the curved space-time around them at the speed of light)。
质量越大的物体,产生的引力波就越大,也就越容易被科学家探测到。
The more massive the object, the larger the wave and the easier for scientists to detect.❺通常引力波的产生非常困难。
地球围绕太阳以每秒30千米的速度前进,发出的引力波功率仅为200瓦,还不如家用电饭煲功率大。
宇宙中大质量天体的加速(accelerate)、碰撞(collide)和合并(coalesce)等事件才可以形成强大的引力波。
专业词汇(天体物理)
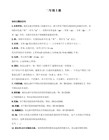
24、blazar spectra blazar光谱
25、polarization 偏振
26、doppler broadened 多普勒展宽
27、碰撞激发 collisional excitation
28、photoionization 光致电离
29、horizon of the universe 宇宙视界
122、termination shock 终端激波
123、very hard binary 甚硬双星
124、weak turbulence theory 弱湍流理论
125、nova-like X-ray source 类新星 X 射线源
126、longitudinal chromatic aberration 纵向色差
87、string theory 宇宙弦理论
88、stripped plasma 全电离等离子体
89、close binary star 密近双星
90、stellar-mass black hole 恒星质量黑洞
91、ultraviolet radiation 紫外辐射
92、aberration 光行差
105、parallax second 秒差距(parsec) (pc)
106、geometric aberration 几何象差
107、electric multipole radiation 电多极辐射
108、Voigt effect 佛克特效应
109、monotonic model 单调宇宙模型
148、fast-spinning black hole 快自旋黑洞
稳定的高功率激光系统在高级引力波探测器中的应用

Stabilized high-power laser system forthe gravitational wave detector advancedLIGOP.Kwee,1,∗C.Bogan,2K.Danzmann,1,2M.Frede,4H.Kim,1P.King,5J.P¨o ld,1O.Puncken,3R.L.Savage,5F.Seifert,5P.Wessels,3L.Winkelmann,3and B.Willke21Max-Planck-Institut f¨u r Gravitationsphysik(Albert-Einstein-Institut),Hannover,Germany2Leibniz Universit¨a t Hannover,Hannover,Germany3Laser Zentrum Hannover e.V.,Hannover,Germany4neoLASE GmbH,Hannover,Germany5LIGO Laboratory,California Institute of Technology,Pasadena,California,USA*patrick.kwee@aei.mpg.deAbstract:An ultra-stable,high-power cw Nd:Y AG laser system,devel-oped for the ground-based gravitational wave detector Advanced LIGO(Laser Interferometer Gravitational-Wave Observatory),was comprehen-sively ser power,frequency,beam pointing and beamquality were simultaneously stabilized using different active and passiveschemes.The output beam,the performance of the stabilization,and thecross-coupling between different stabilization feedback control loops werecharacterized and found to fulfill most design requirements.The employedstabilization schemes and the achieved performance are of relevance tomany high-precision optical experiments.©2012Optical Society of AmericaOCIS codes:(140.3425)Laser stabilization;(120.3180)Interferometry.References and links1.S.Rowan and J.Hough,“Gravitational wave detection by interferometry(ground and space),”Living Rev.Rel-ativity3,1–3(2000).2.P.R.Saulson,Fundamentals of Interferometric Gravitational Wave Detectors(World Scientific,1994).3.G.M.Harry,“Advanced LIGO:the next generation of gravitational wave detectors,”Class.Quantum Grav.27,084006(2010).4. B.Willke,“Stabilized lasers for advanced gravitational wave detectors,”Laser Photon.Rev.4,780–794(2010).5.P.Kwee,“Laser characterization and stabilization for precision interferometry,”Ph.D.thesis,Universit¨a t Han-nover(2010).6.K.Somiya,Y.Chen,S.Kawamura,and N.Mio,“Frequency noise and intensity noise of next-generationgravitational-wave detectors with RF/DC readout schemes,”Phys.Rev.D73,122005(2006).7. B.Willke,P.King,R.Savage,and P.Fritschel,“Pre-stabilized laser design requirements,”internal technicalreport T050036-v4,LIGO Scientific Collaboration(2009).8.L.Winkelmann,O.Puncken,R.Kluzik,C.Veltkamp,P.Kwee,J.Poeld,C.Bogan,B.Willke,M.Frede,J.Neu-mann,P.Wessels,and D.Kracht,“Injection-locked single-frequency laser with an output power of220W,”Appl.Phys.B102,529–538(2011).9.T.J.Kane and R.L.Byer,“Monolithic,unidirectional single-mode Nd:Y AG ring laser,”Opt.Lett.10,65–67(1985).10.I.Freitag,A.T¨u nnermann,and H.Welling,“Power scaling of diode-pumped monolithic Nd:Y AG lasers to outputpowers of several watts,”mun.115,511–515(1995).11.M.Frede,B.Schulz,R.Wilhelm,P.Kwee,F.Seifert,B.Willke,and D.Kracht,“Fundamental mode,single-frequency laser amplifier for gravitational wave detectors,”Opt.Express15,459–465(2007).#161737 - $15.00 USD Received 18 Jan 2012; revised 27 Feb 2012; accepted 4 Mar 2012; published 24 Apr 2012 (C) 2012 OSA7 May 2012 / Vol. 20, No. 10 / OPTICS EXPRESS 1061712. A.D.Farinas,E.K.Gustafson,and R.L.Byer,“Frequency and intensity noise in an injection-locked,solid-statelaser,”J.Opt.Soc.Am.B12,328–334(1995).13.R.Bork,M.Aronsson,D.Barker,J.Batch,J.Heefner,A.Ivanov,R.McCarthy,V.Sandberg,and K.Thorne,“New control and data acquisition system in the Advanced LIGO project,”Proc.of Industrial Control And Large Experimental Physics Control System(ICALEPSC)conference(2011).14.“Experimental physics and industrial control system,”/epics/.15.P.Kwee and B.Willke,“Automatic laser beam characterization of monolithic Nd:Y AG nonplanar ring lasers,”Appl.Opt.47,6022–6032(2008).16.P.Kwee,F.Seifert,B.Willke,and K.Danzmann,“Laser beam quality and pointing measurement with an opticalresonator,”Rev.Sci.Instrum.78,073103(2007).17. A.R¨u diger,R.Schilling,L.Schnupp,W.Winkler,H.Billing,and K.Maischberger,“A mode selector to suppressfluctuations in laser beam geometry,”Opt.Acta28,641–658(1981).18. B.Willke,N.Uehara,E.K.Gustafson,R.L.Byer,P.J.King,S.U.Seel,and R.L.Savage,“Spatial and temporalfiltering of a10-W Nd:Y AG laser with a Fabry-Perot ring-cavity premode cleaner,”Opt.Lett.23,1704–1706 (1998).19.J.H.P¨o ld,“Stabilization of the Advanced LIGO200W laser,”Diploma thesis,Leibniz Universit¨a t Hannover(2009).20. E.D.Black,“An introduction to Pound-Drever-Hall laser frequency stabilization,”Am.J.Phys.69,79–87(2001).21.R.W.P.Drever,J.L.Hall,F.V.Kowalski,J.Hough,G.M.Ford,A.J.Munley,and H.Ward,“Laser phase andfrequency stabilization using an optical resonator,”Appl.Phys.B31,97–105(1983).22. A.Bullington,ntz,M.Fejer,and R.Byer,“Modal frequency degeneracy in thermally loaded optical res-onators,”Appl.Opt.47,2840–2851(2008).23.G.Mueller,“Beam jitter coupling in Advanced LIGO,”Opt.Express13,7118–7132(2005).24.V.Delaubert,N.Treps,ssen,C.C.Harb,C.Fabre,m,and H.-A.Bachor,“TEM10homodynedetection as an optimal small-displacement and tilt-measurement scheme,”Phys.Rev.A74,053823(2006). 25.P.Kwee,B.Willke,and K.Danzmann,“Laser power noise detection at the quantum-noise limit of32A pho-tocurrent,”Opt.Lett.36,3563–3565(2011).26. A.Araya,N.Mio,K.Tsubono,K.Suehiro,S.Telada,M.Ohashi,and M.Fujimoto,“Optical mode cleaner withsuspended mirrors,”Appl.Opt.36,1446–1453(1997).27.P.Kwee,B.Willke,and K.Danzmann,“Shot-noise-limited laser power stabilization with a high-power photodi-ode array,”Opt.Lett.34,2912–2914(2009).28. ntz,P.Fritschel,H.Rong,E.Daw,and G.Gonz´a lez,“Quantum-limited optical phase detection at the10−10rad level,”J.Opt.Soc.Am.A19,91–100(2002).1.IntroductionInterferometric gravitational wave detectors[1,2]perform one of the most precise differential length measurements ever.Their goal is to directly detect the faint signals of gravitational waves emitted by astrophysical sources.The Advanced LIGO(Laser Interferometer Gravitational-Wave Observatory)[3]project is currently installing three second-generation,ground-based detectors at two observatory sites in the USA.The4kilometer-long baseline Michelson inter-ferometers have an anticipated tenfold better sensitivity than theirfirst-generation counterparts (Inital LIGO)and will presumably reach a strain sensitivity between10−24and10−23Hz−1/2.One key technology necessary to reach this extreme sensitivity are ultra-stable high-power laser systems[4,5].A high laser output power is required to reach a high signal-to-quantum-noise ratio,since the effect of quantum noise at high frequencies in the gravitational wave readout is reduced with increasing circulating laser power in the interferometer.In addition to quantum noise,technical laser noise coupling to the gravitational wave channel is a major noise source[6].Thus it is important to reduce the coupling of laser noise,e.g.by optical design or by exploiting symmetries,and to reduce laser noise itself by various active and passive stabilization schemes.In this article,we report on the pre-stabilized laser(PSL)of the Advanced LIGO detector. The PSL is based on a high-power solid-state laser that is comprehensively stabilized.One laser system was set up at the Albert-Einstein-Institute(AEI)in Hannover,Germany,the so called PSL reference system.Another identical PSL has already been installed at one Advanced LIGO site,the one near Livingston,LA,USA,and two more PSLs will be installed at the second #161737 - $15.00 USD Received 18 Jan 2012; revised 27 Feb 2012; accepted 4 Mar 2012; published 24 Apr 2012 (C) 2012 OSA7 May 2012 / Vol. 20, No. 10 / OPTICS EXPRESS 10618site at Hanford,WA,USA.We have characterized the reference PSL and thefirst observatory PSL.For this we measured various beam parameters and noise levels of the output beam in the gravitational wave detection frequency band from about10Hz to10kHz,measured the performance of the active and passive stabilization schemes,and determined upper bounds for the cross coupling between different control loops.At the time of writing the PSL reference system has been operated continuously for more than18months,and continues to operate reliably.The reference system delivered a continuous-wave,single-frequency laser beam at1064nm wavelength with a maximum power of150W with99.5%in the TEM00mode.The active and passive stabilization schemes efficiently re-duced the technical laser noise by several orders of magnitude such that most design require-ments[5,7]were fulfilled.In the gravitational wave detection frequency band the relative power noise was as low as2×10−8Hz−1/2,relative beam pointingfluctuations were as low as1×10−7Hz−1/2,and an in-loop measurement of the frequency noise was consistent with the maximum acceptable frequency noise of about0.1HzHz−1/2.The cross couplings between the control loops were,in general,rather small or at the expected levels.Thus we were able to optimize each loop individually and observed no instabilities due to cross couplings.This stabilized laser system is an indispensable part of Advanced LIGO and fulfilled nearly all design goals concerning the maximum acceptable noise levels of the different beam pa-rameters right after installation.Furthermore all or a subset of the implemented stabilization schemes might be of interest for many other high-precision optical experiments that are limited by laser noise.Besides gravitational wave detectors,stabilized laser systems are used e.g.in the field of optical frequency standards,macroscopic quantum objects,precision spectroscopy and optical traps.In the following section the laser system,the stabilization scheme and the characterization methods are described(Section2).Then,the results of the characterization(Section3)and the conclusions(Section4)are presented.ser system and stabilizationThe PSL consists of the laser,developed and fabricated by Laser Zentrum Hannover e.V.(LZH) and neoLASE,and the stabilization,developed and integrated by AEI.The optical components of the PSL are on a commercial optical table,occupying a space of about1.5×3.5m2,in a clean,dust-free environment.At the observatory sites the optical table is located in an acoustically isolated cleanroom.Most of the required electronics,the laser diodes for pumping the laser,and water chillers for cooling components on the optical table are placed outside of this cleanroom.The laser itself consists of three stages(Fig.1).An almostfinal version of the laser,the so-called engineering prototype,is described in detail in[8].The primary focus of this article is the stabilization and characterization of the PSL.Thus only a rough overview of the laser and the minor modifications implemented between engineering prototype and reference system are given in the following.Thefirst stage,the master laser,is a commercial non-planar ring-oscillator[9,10](NPRO) manufactured by InnoLight GmbH in Hannover,Germany.This solid-state laser uses a Nd:Y AG crystal as the laser medium and resonator at the same time.The NPRO is pumped by laser diodes at808nm and delivers an output power of2W.An internal power stabilization,called the noise eater,suppresses the relaxation oscillation at around1MHz.Due to its monolithic res-onator,the laser has exceptional intrinsic frequency stability.The two subsequent laser stages, used for power scaling,inherit the frequency stability of the master laser.The second stage(medium-power amplifier)is a single-pass amplifier[11]with an output power of35W.The seed laser beam from the NPRO stage passes through four Nd:YVO4crys-#161737 - $15.00 USD Received 18 Jan 2012; revised 27 Feb 2012; accepted 4 Mar 2012; published 24 Apr 2012 (C) 2012 OSA7 May 2012 / Vol. 20, No. 10 / OPTICS EXPRESS 10619power stabilizationFig.1.Pre-stabilized laser system of Advanced LIGO.The three-staged laser(NPRO,medium power amplifier,high power oscillator)and the stabilization scheme(pre-mode-cleaner,power and frequency stabilization)are shown.The input-mode-cleaner is not partof the PSL but closely related.NPRO,non-planar ring oscillator;EOM,electro-optic mod-ulator;FI,Faraday isolator;AOM,acousto-optic modulator.tals which are longitudinally pumped byfiber-coupled laser diodes at808nm.The third stage is an injection-locked ring oscillator[8]with an output power of about220W, called the high-power oscillator(HPO).Four Nd:Y AG crystals are used as the active media. Each is longitudinally pumped by sevenfiber-coupled laser diodes at808nm.The oscillator is injection-locked[12]to the previous laser stage using a feedback control loop.A broadband EOM(electro-optic modulator)placed between the NPRO and the medium-power amplifier is used to generate the required phase modulation sidebands at35.5MHz.Thus the high output power and good beam quality of this last stage is combined with the good frequency stability of the previous stages.The reference system features some minor modifications compared to the engineering proto-type[8]concerning the optics:The external halo aperture was integrated into the laser system permanently improving the beam quality.Additionally,a few minor designflaws related to the mechanical structure and the optical layout were engineered out.This did not degrade the output performance,nor the characteristics of the locked laser.In general the PSL is designed to be operated in two different power modes.In high-power mode all three laser stages are engaged with a power of about160W at the PSL output.In low-power mode the high-power oscillator is turned off and a shutter inside the laser resonator is closed.The beam of the medium-power stage is reflected at the output coupler of the high power stage leaving a residual power of about13W at the PSL output.This low-power mode will be used in the early commissioning phase and in the low-frequency-optimized operation mode of Advanced LIGO and is not discussed further in this article.The stabilization has three sections(Fig.1:PMC,PD2,reference cavity):A passive resonator, the so called pre-mode-cleaner(PMC),is used tofilter the laser beam spatially and temporally (see subsection2.1).Two pick-off beams at the PMC are used for the active power stabilization (see subsection2.2)and the active frequency pre-stabilization,respectively(see subsection2.3).In general most stabilization feedback control loops of the PSL are implemented using analog electronics.A real-time computer system(Control and Data Acquisition Systems,CDS,[13]) which is common to many other subsystems of Advanced LIGO,is utilized to control and mon-itor important parameters of the analog electronics.The lock acquisition of various loops,a few #161737 - $15.00 USD Received 18 Jan 2012; revised 27 Feb 2012; accepted 4 Mar 2012; published 24 Apr 2012 (C) 2012 OSA7 May 2012 / Vol. 20, No. 10 / OPTICS EXPRESS 10620slow digital control loops,and the data acquisition are implemented using this computer sys-tem.Many signals are recorded at different sampling rates ranging from16Hz to33kHz for diagnostics,monitoring and vetoing of gravitational wave signals.In total four real-time pro-cesses are used to control different aspects of the laser system.The Experimental Physics and Industrial Control System(EPICS)[14]and its associated user tools are used to communicate with the real-time software modules.The PSL contains a permanent,dedicated diagnostic instrument,the so called diagnostic breadboard(DBB,not shown in Fig.1)[15].This instrument is used to analyze two different beams,pick-off beams of the medium power stage and of the HPO.Two shutters are used to multiplex these to the DBB.We are able to measurefluctuations in power,frequency and beam pointing in an automated way with this instrument.In addition the beam quality quantified by the higher order mode content of the beam was measured using a modescan technique[16].The DBB is controlled by one real-time process of the CDS.In contrast to most of the other control loops in the PSL,all DBB control loops were implemented digitally.We used this instrument during the characterization of the laser system to measure the mentioned laser beam parameters of the HPO.In addition we temporarily placed an identical copy of the DBB downstream of the PMC to characterize the output beam of the PSL reference system.2.1.Pre-mode-cleanerA key component of the stabilization scheme is the passive ring resonator,called the pre-mode-cleaner(PMC)[17,18].It functions to suppress higher-order transverse modes,to improve the beam quality and the pointing stability of the laser beam,and tofilter powerfluctuations at radio frequencies.The beam transmitted through this resonator is the output beam of the PSL, and it is delivered to the subsequent subsystems of the gravitational wave detector.We developed and used a computer program[19]to model thefilter effects of the PMC as a function of various resonator parameters in order to aid its design.This led to a resonator with a bow-tie configuration consisting of four low-loss mirrors glued to an aluminum spacer. The optical round-trip length is2m with a free spectral range(FSR)of150MHz.The inci-dence angle of the horizontally polarized laser beam is6◦.Theflat input and output coupling mirrors have a power transmission of2.4%and the two concave high reflectivity mirrors(3m radius of curvature)have a transmission of68ppm.The measured bandwidth was,as expected, 560kHz which corresponds to afinesse of133and a power build-up factor of42.The Gaussian input/output beam had a waist radius of about568µm and the measured acquired round-trip Gouy phase was about1.7rad which is equivalent to0.27FSR.One TEM00resonance frequency of the PMC is stabilized to the laser frequency.The Pound-Drever-Hall(PDH)[20,21]sensing scheme is used to generate error signals,reusing the phase modulation sidebands at35.5MHz created between NPRO and medium power amplifier for the injection locking.The signal of the photodetector PD1,placed in reflection of the PMC, is demodulated at35.5MHz.This photodetector consists of a1mm InGaAs photodiode and a transimpedance amplifier.A piezo-electric element(PZT)between one of the curved mirrors and the spacer is used as a fast actuator to control the round-trip length and thereby the reso-nance frequencies of the PMC.With a maximum voltage of382V we were able to change the round-trip length by about2.4µm.An analog feedback control loop with a bandwidth of about 7kHz is used to stabilize the PMC resonance frequency to the laser frequency.In addition,the electronics is able to automatically bring the PMC into resonance with the laser(lock acquisition).For this process a125ms period ramp signal with an amplitude cor-responding to about one FSR is applied to the PZT of the PMC.The average power on pho-todetector PD1is monitored and as soon as the power drops below a given threshold the logic considers the PMC as resonant and closes the analog control loop.This lock acquisition proce-#161737 - $15.00 USD Received 18 Jan 2012; revised 27 Feb 2012; accepted 4 Mar 2012; published 24 Apr 2012 (C) 2012 OSA7 May 2012 / Vol. 20, No. 10 / OPTICS EXPRESS 10621dure took an average of about65ms and is automatically repeated as soon as the PMC goes off resonance.One real-time process of CDS is dedicated to control the PMC electronics.This includes parameters such as the proportional gain of the loop or lock acquisition parameters.In addition to the PZT actuator,two heating foils,delivering a maximum total heating power of14W,are attached to the aluminum spacer to control its temperature and thereby the roundtrip length on timescales longer than3s.We measured a heating and cooling1/e time constant of about2h with a range of4.5K which corresponds to about197FSR.During maintenance periods we heat the spacer with7W to reach a spacer temperature of about2.3K above room temperature in order to optimize the dynamic range of this actuator.A digital control loop uses this heater as an actuator to off-load the PZT actuator allowing compensation for slow room temperature and laser frequency drifts.The PMC is placed inside a pressure-tight tank at atmospheric pressure for acoustic shield-ing,to avoid contamination of the resonator mirrors and to minimize optical path length changes induced by atmospheric pressure variations.We used only low-outgassing materials and fabri-cated the PMC in a cleanroom in order to keep the initial mirror contamination to a minimum and to sustain a high long-term throughput.The PMCfilters the laser beam and improves the beam quality of the laser by suppress-ing higher order transverse modes[17].The acquired round-trip Gouy phase of the PMC was chosen in such a way that the resonance frequencies of higher order TEM modes are clearly separated from the TEM00resonance frequency.Thus these modes are not resonant and are mainly reflected by the PMC,whereas the TEM00mode is transmitted.However,during the design phase we underestimated the thermal effects in the PMC such that at nominal circu-lating power the round-trip Gouy-phase is close to0.25FSR and the resonance of the TEM40 mode is close to that of the TEM00mode.To characterize the mode-cleaning performance we measured the beam quality upstream and downstream of the PMC with the two independent DBBs.At150W in the transmitted beam,the circulating power in the PMC is about6.4kW and the intensity at the mirror surface can be as high as1.8×1010W m−2.At these power levels even small absorptions in the mirror coatings cause thermal effects which slightly change the mirror curvature[22].To estimate these thermal effects we analyzed the transmitted beam as a function of the circulating power using the DBB.In particular we measured the mode content of the LG10and TEM40mode.Changes of the PMC eigenmode waist size showed up as variations of the LG10mode content.A power dependence of the round-trip Gouy phase caused a variation of the power within the TEM40mode since its resonance frequency is close to a TEM00mode resonance and thus the suppression of this mode depends strongly on the Gouy phase.We adjusted the input power to the PMC such that the transmitted power ranged from100W to 150W corresponding to a circulating power between4.2kW and6.4kW.We used our PMC computer simulation to deduce the power dependence of the eigenmode waist size and the round-trip Gouy phase.The results are given in section3.1.At all circulating power levels,however,the TEM10and TEM01modes are strongly sup-pressed by the PMC and thus beam pointingfluctuations are reduced.Pointingfluctuations can be expressed tofirst order as powerfluctuations of the TEM10and TEM01modes[23,24].The PMC reduces thefield amplitude of these modes and thus the pointingfluctuations by a factor of about61according to the measuredfinesse and round-trip Gouy phase.To keep beam point-ingfluctuations small is important since they couple to the gravitational wave channel by small differential misalignments of the interferometer optics.Thus stringent design requirements,at the10−6Hz−1/2level for relative pointing,were set.To verify the pointing suppression effect of the PMC we used DBBs to measure the beam pointingfluctuations upstream and downstream #161737 - $15.00 USD Received 18 Jan 2012; revised 27 Feb 2012; accepted 4 Mar 2012; published 24 Apr 2012 (C) 2012 OSA7 May 2012 / Vol. 20, No. 10 / OPTICS EXPRESS 10622Fig.2.Detailed schematic of the power noise sensor setup for thefirst power stabilizationloop.This setup corresponds to PD2in the overview in Fig.1.λ/2,waveplate;PBS,polar-izing beam splitter;BD,glassfilters used as beam dump;PD,single element photodetector;QPD,quadrant photodetector.of the PMC.The resonator design has an even number of nearly normal-incidence reflections.Thus the resonance frequencies of horizontal and vertical polarized light are almost identical and the PMC does not act as polarizer.Therefore we use a thin-film polarizer upstream of the PMC to reach the required purity of larger than100:1in horizontal polarization.Finally the PMC reduces technical powerfluctuations at radio frequencies(RF).A good power stability between9MHz and100MHz is necessary as the phase modulated light in-jected into the interferometer is used to sense several degrees of freedom of the interferometer that need to be controlled.Power noise around these phase modulation sidebands would be a noise source for the respective stabilization loop.The PMC has a bandwidth(HWHM)of about 560kHz and acts tofirst order as a low-passfilter for powerfluctuations with a-3dB corner frequency at this frequency.To verify that the suppression of RF powerfluctuations is suffi-cient to fulfill the design requirements,we measured the relative power noise up to100MHz downstream of the PMC with a dedicated experiment involving the optical ac coupling tech-nique[25].In addition the PMC serves the very important purpose of defining the spatial laser mode for the downstream subsystem,namely the input optics(IO)subsystem.The IO subsystem is responsible,among other things,to further stabilize the laser beam with the suspended input mode cleaner[26]before the beam will be injected into the interferometer.Modifications of beam alignment or beam size of the laser system,which were and might be unavoidable,e.g., due to maintenance,do not propagate downstream of the PMC tofirst order due to its mode-cleaning effect.Furthermore we benefit from a similar isolating effect for the active power and frequency stabilization by using the beams transmitted through the curved high-reflectivity mirrors of the PMC.2.2.Power stabilizationThe passivefiltering effect of the PMC reduces powerfluctuations significantly only above the PMC bandwidth.In the detection band from about10Hz to10kHz good power stability is required sincefluctuations couple via the radiation pressure imbalance and the dark-fringe offset to the gravitational wave channel.Thus two cascaded active control loops,thefirst and second power stabilization loop,are used to reduce powerfluctuations which are mainly caused by the HPO stage.Thefirst loop uses a low-noise photodetector(PD2,see Figs.1and2)at one pick-off port #161737 - $15.00 USD Received 18 Jan 2012; revised 27 Feb 2012; accepted 4 Mar 2012; published 24 Apr 2012 (C) 2012 OSA7 May 2012 / Vol. 20, No. 10 / OPTICS EXPRESS 10623of the PMC to measure the powerfluctuations downstream of the PMC.An analog electronics feedback control loop and an AOM(acousto-optic modulator)as actuator,located upstream of the PMC,are used to stabilize the power.Scattered light turned out to be a critical noise source for thisfirst loop.Thus we placed all required optical and opto-electronic components into a box to shield from scattered light(see Fig.2).The beam transmitted by the curved PMC mirror has a power of about360mW.This beam isfirst attenuated in the box using aλ/2waveplate and a thin-film polarizer,such that we are able to adjust the power on the photodetectors to the optimal operation point.Afterwards the beam is split by a50:50beam splitter.The beams are directed to two identical photode-tectors,one for the control loop(PD2a,in-loop detector)and one for independent out-of-loop measurements to verify the achieved power stability(PD2b,out-of-loop detector).These pho-todetectors consist of a2mm InGaAs photodiode(PerkinElmer C30642GH),a transimpedance amplifier and an integrated signal-conditioningfilter.At the chosen operation point a power of about4mW illuminates each photodetector generating a photocurrent of about3mA.Thus the shot noise is at a relative power noise of10−8Hz−1/2.The signal conditioningfilter has a gain of0.2at very low frequencies(<70mHz)and amplifies the photodetector signal in the im-portant frequency range between3.3Hz and120Hz by about52dB.This signal conditioning filter reduces the electronics noise requirements on all subsequent stages,but has the drawback that the range between3.3Hz and120Hz is limited to maximum peak-to-peak relative power fluctuations of5×10−3.Thus the signal-conditioned channel is in its designed operation range only when the power stabilization loop is closed and therefore it is not possible to measure the free running power noise using this channel due to saturation.The uncoated glass windows of the photodiodes were removed and the laser beam hits the photodiodes at an incidence angle of45◦.The residual reflection from the photodiode surface is dumped into a glassfilter(Schott BG39)at the Brewster angle.Beam positionfluctuations in combination with spatial inhomogeneities in the photodiode responsivity is another noise source for the power stabilization.We placed a silicon quadrant photodetector(QPD)in the box to measure the beam positionfluctuations of a low-power beam picked off the main beam in the box.The beam parameters,in particular the Gouy phase,at the QPD are the same as on the power sensing detectors.Thus the beam positionfluctuations measured with the QPD are the same as the ones on the power sensing photodetectors,assuming that the positionfluctuations are caused upstream of the QPD pick-off point.We used the QPD to measure beam positionfluctuations only for diagnostic and noise projection purposes.In a slightly modified experiment,we replaced one turning mirror in the path to the power sta-bilization box by a mirror attached to a tip/tilt PZT element.We measured the typical coupling between beam positionfluctuations generated by the PZT and the residual relative photocurrent fluctuations measured with the out-of-the-loop photodetector.This coupling was between1m−1 and10m−1which is a typical value observed in different power stabilization experiments as well.We measured this coupling factor to be able to calculate the noise contribution in the out-of-the-loop photodetector signal due to beam positionfluctuations(see Subsection3.3).Since this tip/tilt actuator was only temporarily in the setup,we are not able to measure the coupling on a regular basis.Both power sensing photodetectors are connected to analog feedback control electronics.A low-pass(100mHz corner frequency)filtered reference value is subtracted from one signal which is subsequently passed through several control loopfilter stages.With power stabilization activated,we are able to control the power on the photodetectors and thereby the PSL output power via the reference level on time scales longer than10s.The reference level and other important parameters of these electronics are controlled by one dedicated real-time process of the CDS.The actuation or control signal of the electronics is passed to an AOM driver #161737 - $15.00 USD Received 18 Jan 2012; revised 27 Feb 2012; accepted 4 Mar 2012; published 24 Apr 2012 (C) 2012 OSA7 May 2012 / Vol. 20, No. 10 / OPTICS EXPRESS 10624。
Einstein-Rosen, On Gravitational Waves(1937)
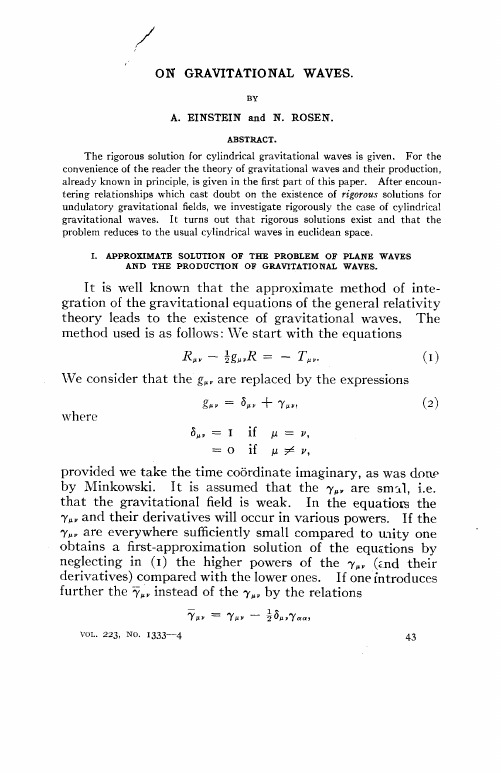
We consider t h a t the g., are replaced by the expressions where
g., = a., + u.,,
~,v = I
(2)
=o
if if
/~ = v. ~¢v,
provided we take the time co6rdinate imaginary, as was dotLo by Minkowski. It is assumed t h a t the %. are smal, i.e. t h a t the gravitational field is weak. In the equatiors the 3`.. and their derivatives will occur in various powers. If the 3`.. are everywhere sufficiently small compared to uaity one obtains a first-approximation solution of the equations by neglecting in (I) the higher powers of the 3`.. (~nd their derivatives) compared with the lower ones. If one introduces further the ~., instead of the 3`.. by the relations
/
ON GRAVITATIONAL WAVES.
BY A. E I N S T E I N and N. R O S E N .
[指南]专业词汇(天体物理)
![[指南]专业词汇(天体物理)](https://img.taocdn.com/s3/m/28d39093bdeb19e8b8f67c1cfad6195f312be84a.png)
专业词汇(天体物理)1、Galactic background γ-ray radiation 银河γ背景辐射2、active galactic nuclei 活动星系核3、radio survey 射电巡天4、ultraviolet fluxes 紫外流量5、Roche-lobe overflow 洛希瓣超流6、abnormal redshift 反常红移7、absorption cross section 吸收截面8、acceleration mechanism 加速机制9、general electric synchrotron 广义电同步加速10、screening effect 屏蔽效应11、visible spectrum 可见光谱12、celestial matter 宇宙物质13、broad emission-line 宽发射线14、narrow emission-line 窄发射线15、planetary nebulae 行星状星云16、isotropic antenna 各向同性天线17、Seyfert galaxy 赛弗特星系18、ultraviolet continuum 紫外连续谱19、bolometric luminosity 光度20、variable time scale 可变时标21、continuum emission 连续辐射22、power law 幂率23、non-thermal 非热24、blazar spectra blazar光谱25、polarization 偏振26、doppler broadened 多普勒展宽27、碰撞激发 collisional excitation28、photoionization 光致电离29、horizon of the universe 宇宙视界30、Coulomb collision 库仑碰撞31、Coulomb coupling 库仑耦合32、Born approximation 玻恩近似33、absolute magnitude 绝对星等34、absolute parallax 绝对视差35、overmassive object 超大质量天体36、gravitational wave 引力波37、gravitational-wave astronomy 引力波天文学38、error distribution 误差分布39、chromatic aberration 色差40、characteristic function 特征函数41、CGRO 康普顿γ射线天文台(Compton Gamma-Ray Observatory)42、central lobe 中心瓣43、Rayleigh criterion 瑞利判据44、Rayleigh limit 瑞利极限45、quasi-stellar object 类星体(QSO)46、quiescent radiation 宁静辐射47、quiescent spectrum 宁静光谱48、radial-velocity survey 视向速度巡天49、absorption frequency 吸收频率50、background radiation 背景辐射51、light illumination 光照度52、radiant power 辐射功率53、anisotropic medium 各向异性介质54、plasma jet instability 各向异性等离子体喷流不稳定性55、cosmic rays 宇宙线56、Zeeman effect 塞曼效应57、annihilation radiation 湮灭辐射58、radiometric magnitude 辐射星等59、proton flux 质子流量60、Abell cluster 阿贝尔星系团61、autocorrelation coefficient 自相关系数62、dark matter 暗物质63、Maxwellion distribution 麦克斯韦分布64、black hole binary 黑洞双星65、blanketing factor 覆盖因子66、radio galaxy 射电星系67、Cauchy's dispersion formula 柯西色散公式68、intergalactic medium 星系际介质69、shock wave 激波70、background Compton scattering 背景康普顿散射71、dark energy 暗能量72、multiple galaxy 多重星系73、current density 流密度74、thermodynamic equilibrium 热动平衡75、thermal excitation 热激发76、synchrotron radiation 同步加速辐射77、synchro-cyclotron radiation 同步-回旋加速辐射78、center of curvature 曲率中心79、super-relativistic effect 极端相对论性效应80、active binary 活动双星81、compact binary 致密双星82、compact galaxy nucleus 致密星系核83、supernova explosion 超新星爆发84、supernova ejecta 超新星喷射物85、deflection angle 偏转角86、cosmic noise absorption 宇宙噪声吸收87、string theory 宇宙弦理论88、stripped plasma 全电离等离子体89、close binary star 密近双星90、stellar-mass black hole 恒星质量黑洞91、ultraviolet radiation 紫外辐射92、aberration 光行差93、spontaneous emission 自发发射94、curvature radiation 曲率辐射95、spontaneous transition 自发跃迁96、peculiar spectrum 特殊光谱97、particle horizon 粒子视界98、stimulated radiation 受激辐射99、stimulated emission 受激发射100、circular polarization 圆偏振101、damping radiation 阻尼辐射102、spherical harmonics 球谐函数103、parity nonconservation 宇称不守恒104、drifting zebras 漂移带105、parallax second 秒差距(parsec) (pc)106、geometric aberration 几何象差107、electric multipole radiation 电多极辐射108、Voigt effect 佛克特效应109、monotonic model 单调宇宙模型110、isochronous correspondence 等时对应111、gas nebula 气体星云112、cepheid parallax 造父视差113、diffusion equation 扩散方程114、advancing shock front 前进激波前115、advection dominated accretion 径移吸积流116、spherical potential 球对称势117、luminous emittance 发光度118、cosmic gusher 宇宙喷射源119、microwave background 微波背景120、herpolhode 空间极迹121、heat of desorption 退吸热122、termination shock 终端激波123、very hard binary 甚硬双星124、weak turbulence theory 弱湍流理论125、nova-like X-ray source 类新星 X 射线源126、longitudinal chromatic aberration 纵向色差127、statistical equilibrium 统计平衡128、Compton cross-section 康普顿截面129、irregular nebula 不规则星云130、stellar astrophysics 恒星天体物理131、background radiation intensity 背景辐射强度132、optical binary 光学双星133、astronomical coordinate system 天文坐标系统134、orbital inclination 轨道倾角135、critical mass 临界质量136、Kerr-Newman black hole 克尔-纽曼黑洞137、Kerr-Newman metric 克尔-纽曼度规138、chromatism 色差139、reflection at critical angle 临界角反射140、source brightness distribution 源亮度分布141、Laplace's nebular hypothesis 拉普拉斯星云假说142、photoionized plasma 光电离等离子体143、photoluminescence 光致发光144、relaxation time 弛豫时间145、cross-correlation function 互相关函数146、relaxed cluster 驰豫星团147、disk-like structure 盘状结构148、fast-spinning black hole 快自旋黑洞149、Fermi Gamma-ray Space Telescope 费米γ射线空间望远镜150、time reversal 时间反演。
怀疑与坚持从引力波的发现谈起作文

怀疑与坚持从引力波的发现谈起作文英文回答:The discovery of gravitational waves was a groundbreaking scientific achievement that has sparked both skepticism and persistence in the scientific community. Gravitational waves were first predicted by Albert Einstein in his theory of general relativity over a century ago, but it was not until 2015 that they were directly observed for the first time.The skepticism surrounding the discovery ofgravitational waves primarily stems from the fact that they are incredibly faint and difficult to detect. Gravitational waves are ripples in the fabric of spacetime caused by the acceleration of massive objects, such as black holes or neutron stars. These waves propagate through the universe, carrying information about the objects that created them. However, the effects of gravitational waves on spacetimeare minuscule, making their detection extremely challenging.Despite the skepticism, scientists persisted in their search for gravitational waves using advanced technology and innovative techniques. The Laser Interferometer Gravitational-Wave Observatory (LIGO) played a crucial role in the discovery. LIGO consists of two identical detectors located in different parts of the United States. Each detector consists of two perpendicular arms with mirrors at the ends. When a gravitational wave passes through the detectors, it causes the arms to stretch and compress, altering the path of laser beams that travel through them. By precisely measuring the changes in the laser beams, scientists can detect the presence of gravitational waves.The persistence of scientists paid off when, on September 14, 2015, LIGO detected the first-ever gravitational wave signal. This signal was generated by the merger of two black holes located over a billion light-years away. The detection of gravitational waves provided direct evidence for the existence of black holes and confirmed Einstein's theory of general relativity.中文回答:引力波的发现是一项具有突破性意义的科学成就,引起了科学界的怀疑和坚持。
gravitational wave - 英译汉
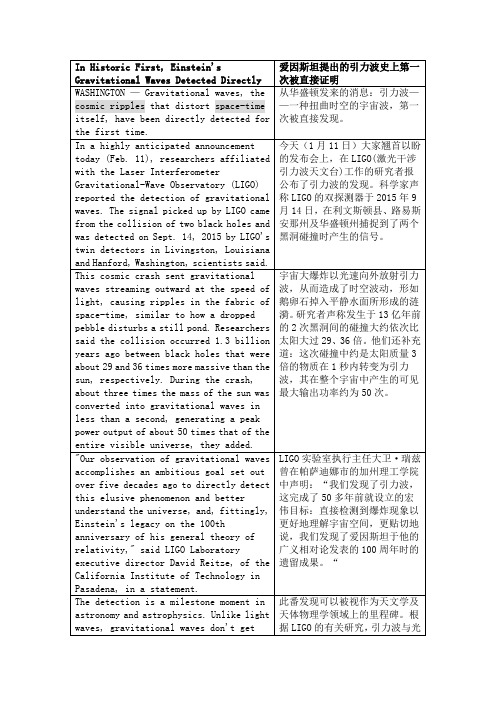
In Historic First, Einstein's Gravitational Waves Detected Directly
爱因斯坦提出的引力波史上第一次被直接证明
WASHINGTON — Gravitational waves, thecosmic ripplesthat distortspace-timeitself, have been directly detected for the first time.
此番发现可以被视作为天文学及天体物理学领域上的里程碑。根据LIGO的有关研究,引力波与光波不同,它们不会在穿越空间地过程中被介质扭曲或者改变;因此能传播有关造成其形成的物质及事件的“纯粹”信息。
Gravitional waves

WWW: /
GRAVITATIONAL WAVES
DANIEL SIGG LIGO Hanford Observatory, P.O. Box 1970 S9-02, Richland, WA 99352, USA E-mail: sigg d@
California Institute of Technology LIGO Project - MS 51-33 Pasadena CA 91125 Phone (626) 395-2129 Fax (626) 304-9834 E-mail: info@
Massachusetts Institute of Technology LIGO Project - MS 20B-145 Cambridge, MA 01239 Phone (617) 253-4824 Fax (617) 253-7014 E-mail: info@
10/23/98
Gravitational Waves
Daniel Sigg LIGO Hanford Observatory, P.O. Box 1970 S9-02, Richland, WA 99352 to be published in the Proceedings of TASI 98 (Theoretical Advanced Study Institute in Elementary Particle Physics) Boulder, Colorado
1
Introduction
According to general relativity theory gravity can be expressed as a spacetime curvature1,2 . One of the theory predictions is that a changing mass distribution can create ripples in space-time which propagate away from the source at the speed of light. These freely propagating ripples in space-time are called gravitational waves. Any attempts to directly detect gravitational waves have not been successful yet. However, their indirect influence has been measured in the binary neutron star system PSR1913+163,4,5,6. This system consist of two neutron stars orbiting each other. One of the neutron stars is active and can be observed as a radio pulsar from earth. Since the observed radio pulses are Doppler shifted by the orbital velocity, the orbital period and its change over time can be determined precisely. If the system behaves according to general relativity theory, it will loose energy through the emission of gravitational waves. As a consequence the two neutron stars will decrease their separation and, thus, orbiting around each other at a higher frequency. From the observed orbital parameters one can first compute the amount of emitted gravitational waves and then the inspiral rate. The calculated and the observed inspiral rates agree within experimental errors (better than 1%). Gravitational waves are quite different from electro-magnetic waves. Most electro-magnetic waves originate from excited atoms and molecules, whereas observable gravitational waves are emitted by accelerated massive objects.
引力波中的相关英语高考考点

引力波中的相关英语高考考点英语可能会在阅读理解中出关于引力波的题目。
相关词汇一定要搞清楚。
引力波 gravitational wave1.由“广义相对论”所预言的“引力子”和“引力波”不存在。
According to the “ general relativity ” predict “ graviton ” and“ gravitational waves ” does not exist.2.因此,高斯束谐振系统对高频遗迹引力波的频率和传播方向具有良好的选择效应。
Therefore, GBRS have a useful selective effect with respect to the frequency and propagation direction of relic HFGWs.3.引力规范理论中的一类引力波方程A Class of Gravitational Waves Equation in Gravitational Gauge Theory4.对物质体系在发射和接收引力波时的能量转换作了新解释.A new interpretation for the energy exchanges of the matter system is given when there exists the gravitational wave.5.谐和条件下的对角度规引力波方程Gravitational Wave Equations under Diagonal Metric and Harmonic Coordinate Conditions6.杨振宁场引力波的极化Polarization of the gravitational waves of yang's gravitational field7.宇宙常数Λ≠0的平面引力波The Plane Gravitational Waves with the Cosmological Constant Λ≠ 08.一种标&张量引力理论的引力波辐射Radiation of gravitational waves in a scalar-tensor theory of gravitation9.De Sitter弯曲时空中遗迹引力波及其能量动量赝张量的表述和正定性问题Relic Gravitational Wave and Positive Definite and Expression of Their Energy-Momentum Pseudo-Tensor in De sitter Background Spacetime of the Curve10.在室内模型激光干涉引力波探测器的基础上,几个野外大型激光干涉引力波探测器正在紧张地建设中。
详解人类首次直接探测到了引力波相关高考考点

详解人类首次直接探测到了引力波相关高考考点美国科学家2016年2月11日宣布,他们探测到引力波的存在。
引力波是爱因斯坦广义相对论实验验证中最后一块缺失的“拼图”。
美国科学家宣布探测到引力波存在新华社华盛顿2月11日电美国科学家11日宣布,他们探测到引力波的存在。
引力波是爱因斯坦广义相对论实验验证中最后一块缺失的“拼图”。
美国加州理工学院、麻省理工学院以及“激光干涉引力波天文台(LIGO)”的研究人员当天在华盛顿举行记者会,宣布他们利用LIGO探测器于2015年9月14日探测到来自于两个黑洞合并的引力波信号。
据他们估计,这两个黑洞合并前的质量分别相当于36个与29个太阳质量,合并后的总质量是62个太阳质量,其中相当于3个太阳质量的能量在合并过程中以引力波的形式释放。
LIGO探测器是美国分别在路易斯安那州利文斯顿市与华盛顿州小城汉福德市建造的两个引力波探测器,不久前完成了改造升级,其探测灵敏度相比2010年提高了约10倍。
引力波是爱因斯坦广义相对论实验验证中最后一块缺失的拼图,专家称这有助于人类揭开宇宙的各种谜团,甚至了解宇宙的开端和运行机制。
这是一个划时代的发现,对于全人类都有重大意义!更有不少中国考生调侃这一发现称:我看见了今年的高考语文题,高考地理题,高考英语题,高考历史题,高考政治题,高考物理题……考查形式很多,以下列出部分相关考点,供大家参考。
·语文会怎么考引力波?【2012年北京卷】在文中横线出填入下列语句,衔接恰当的一项时是()如果有黑洞撞向地球,那么_______。
当然,你听到的不是声波,而是引力波,因为_______。
当黑洞靠近时,引力波会“挤压”内耳骨,产生类似照相机闪光灯充电是发出的咝咝声。
尽管天文学家认为,_____,但正常情况下,_____。
①引力波每时每刻都在影响着我们②你会听到它悄然逼近的声音③引力波是听不到④声波在真空中无法传播A.②③①④B.②④①③C.③②①④D.③①④②答案解析:根据前后文,特别是”正常情况下“这个词,可以确定末项只能为③!答案唯一!为B!另外,看第二空也可以一步搞定!【传统方法】此题先看选项,第一空只有②③两种可能,比较之下,必然选②,排除C、D。
引力波通达信 原理
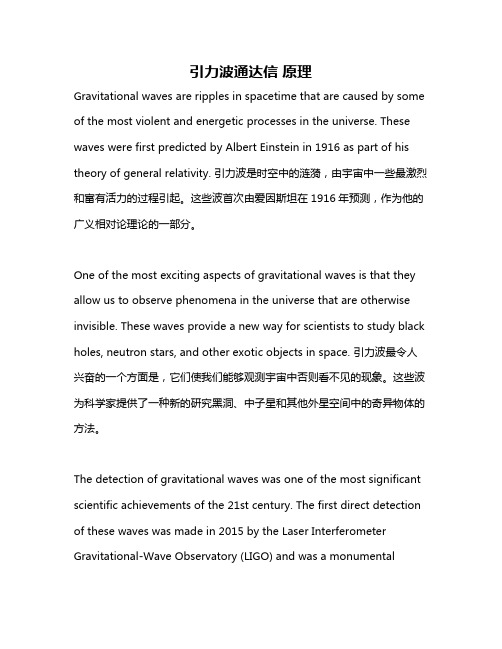
引力波通达信原理Gravitational waves are ripples in spacetime that are caused by some of the most violent and energetic processes in the universe. These waves were first predicted by Albert Einstein in 1916 as part of his theory of general relativity. 引力波是时空中的涟漪,由宇宙中一些最激烈和富有活力的过程引起。
这些波首次由爱因斯坦在1916年预测,作为他的广义相对论理论的一部分。
One of the most exciting aspects of gravitational waves is that they allow us to observe phenomena in the universe that are otherwise invisible. These waves provide a new way for scientists to study black holes, neutron stars, and other exotic objects in space. 引力波最令人兴奋的一个方面是,它们使我们能够观测宇宙中否则看不见的现象。
这些波为科学家提供了一种新的研究黑洞、中子星和其他外星空间中的奇异物体的方法。
The detection of gravitational waves was one of the most significant scientific achievements of the 21st century. The first direct detection of these waves was made in 2015 by the Laser Interferometer Gravitational-Wave Observatory (LIGO) and was a monumentalmoment for astrophysics. 引力波的探测是21世纪最重大的科学成就之一。
Cosmic String Loops and Gravitational Radiaton宇宙弦圈与引力辐射 共46页
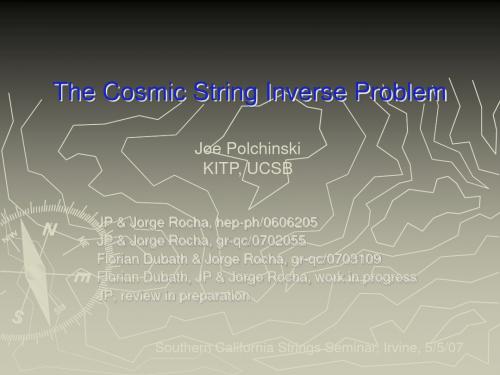
2. Expansion
FRW metric: String EOM:
gauge L/R form: define unit vectors: Then:
3. Long string intercommutation
Produces L- and R-moving kinks. Expansion of the universe straightens these slowly, but more enter the horizon (BB).
direction wrapped. • Solitonic strings and branes in ten dimensions • Solitons involving compactification moduli • Magnetic flux tubes (classical solitons) in the effective
• What are the current bounds, and prospects for improvement?
• Too what extent can we distinguish different kinds of cosmic string?
• What are the current bounds, and prospects for improvement?
If so, simulations (Albrecht & Turok, Bennett & Bouchet, Allen & Shellard, ~ 1989) would have readily given a quantitative understanding.
However, one sees kinks and loops on shorter scales (BB). Limitations: UV cutoff, expansion time.
- 1、下载文档前请自行甄别文档内容的完整性,平台不提供额外的编辑、内容补充、找答案等附加服务。
- 2、"仅部分预览"的文档,不可在线预览部分如存在完整性等问题,可反馈申请退款(可完整预览的文档不适用该条件!)。
- 3、如文档侵犯您的权益,请联系客服反馈,我们会尽快为您处理(人工客服工作时间:9:00-18:30)。
Einstein field equation
2017 NOBEL PRIZE IN PHYSICS
• Reina weiss’s laser interferometer gravitational wave detector is the basis of LIGO devices. He first analyzed the main noise source of the detector and led the research of LIGO instrument science, which eventually made LIGO achieve sufficient sensitivity. • Kip thorne , laid the foundation of gravitational wave detection theory, he created the research direction of gravitational wave calculation and data analysis, and made an important contribution to LIGO instrument science, especially put forward a series of basic concepts of quantum metrology theory. • Barry barish , led the LIGO construction and initial operation, established the LIGO scientific collaboration international, he put the LIGO from several small scientific research team engaged in successfully transformed in many members of the big science and rely on large-scale equipment, eventually make gravitational waves to detect possible.
PHYSICS CAN BE REVOLUTIONARY
From the progress of proving the existence of the gravitational waves , we can have a better understanding of the beauty and power of phsyics . Because the high-tech astronomical telescope must be with the help of light , we cannot see the black hole . But now ,by means of the gravitational wave ,we can hear the black hole . Now the team of LIGO has give us a powerful weapon to explore a new world . That is why people is supposed to work on physics.
• To achieve the joint observation of electromagnetic spectrum.
• To expand the frequency coverage of gravitational wave detection. • To take the observation of gravitational wave events beyond binary satellites. • To provide the standardized cosmological independent candle so that the gravitational wave can be a good tool in cosmpredicted that these distortions could travel across the Universe stretching and squeezing space and time as they move. And this is what we call gravitational waves like ripples
What is more ,proving the existence of the gravitational wave can be a sign of people’s intelligence . Albert Einstein Put forward the concept half a century ago .Now , Rai Weiss has confirmed it. Rai Weiss’s effort has shown the greatness of people’s mind . We can see what we can’t see using our eyes by means of our mind . And we can predict what will happen in the far distant place.
TO HUSTERS
We can learn that the great effort spent on the science will finally pay us off . So if you choose the path of scientific research ,we may be fed up and exhausted . But what you have thought of or founded can be a great inspiration to others and turn into some more important discovery .What you have done may be appreciated in the future. If you major in engineering and work on some specific problems ,you can still be proud of being one of the great human beings . And we can also make the earth a better place to live .We have an equivalent chance of going down in history.
• In Einstein‘s theory of general relativity, gravity is treated as a phenomenon resulting from the curvature of spacetime. This curvature is caused by the presence of mass. Generally, the more mass that is contained within a given volume of space, the greater the curvature of spacetime will be at the boundary of its volume。As objects with mass move around in spacetime, the curvature changes to reflect the changed locations of those objects. In certain circumstances, accelerating objects generate changes in this curvature, which propagate outwards at the speed of light in a wave-like manner. These propagating phenomena are known as gravitational waves.
WHAT GRAVITATIONAL WAVE CAN DO
• To continue to improve the sensitivity is helpful in testing black hole physics. • To build Global Networking to improve the response ability of different directions and the positioning mode.
DEFINITION
• Einstein hypothesized that all objects with mass would distort the fabric of space-time lick a bowling bowl on trampoline.
If their mass is great enough, those distortions could cause other objects to fall into them. This is what we call gravity. For example , the reason why the earth goes around son is that the sun is very massive