Effects_of_Soil_Water_Content_on_Cotton_Root_Growth_and_Distribution_Under_Mulched_Drip_Irrigation1
网络世界2011年秋季版:地面和天气说明书
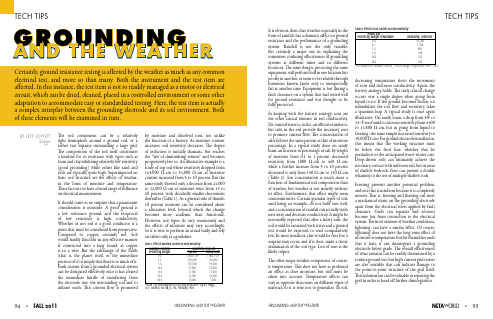
GroundinGand the WeatherThe soil component can be a relatively tight hemisphere around a ground rod, or a rather vast expanse surrounding a large grid. The composition of the soil itself constitutes a baseline for its resistance with types such as loam and clay exhibiting relatively low resistivity (good grounding) while others like sand and rock are typically quite high. Superimposed on basic soil structure are the effects of weather, in the form of moisture and temperature. These factors too have a broad range of influence on electrical measurement.It should come as no surprise that a paramount consideration is moisture. A good ground is a low resistance ground, and the reciprocal of low resistance is high conductivity. Whether or not soil is a good conductor is a point that must be considered from perspective. Compared to copper, certainly not. Soil would hardly function in any effective mannerif constricted into a long strand, as copper is in a wire. But the advantage of the Earth (that is, the planet itself, or the immediateportion of it) is simply that there is so much of it. Fault current from a grounded electrical system can be dissipated effectively once it has cleared the immediate hurdle of transferring from the electrode into the surrounding soil and to infinite earth. This current flow is promoted by moisture and dissolved ions, not unlike the function of a battery. As moisture content increases, soil resistivity decreases. The degree of reduction is initially dramatic, but reaches the “law of diminishing returns” and becomes progressively less so. An illustrative example is a study of top soil where resistivity dropped from 165,000 Ω-cm to 53,000 Ω-cm as moisture content increased from 5 to 10 percent. But the same study showed only a decrease from 21,000 to 12,000 Ω-cm as moisture went from 15 to 20 percent, with decidedly smaller decrements thereafter (Table 1). As a general rule of thumb,18 percent moisture can be considered about a saturation level, beyond which the change becomes more academic than functional. However, soil types do vary enormously and the effects of moisture may vary accordingly. So it is wise to perform an actual study and rely on tables only as a guideline.BY JEFF JOWETTMeggerTECH TIPS Certainly, ground resistance testing is affected by the weather as much as any common electrical test, and more so than many. Both the instrument and the test item are affected. In this instance, the test item is not so readily managed as a motor or electrical circuit, which can be dried, cleaned, placed in a controlled environment or some other adaptation to accommodate easy or standardized testing. Here, the test item is actually a complex interplay between the grounding electrode and its soil environment. Both of these elements will be examined in turn.It is obvious, then, that weather, especially in the form of rainfall, has a dramatic effect on ground resistance and the performance of a grounding system. Rainfall is not the only variable, but certainly a major one in explaining the sometimes confusing effectiveness of grounding systems at different times and in different locations. The same design, protecting the same equipment, will perform well in one location but poorly in another, or seem to be reliable through numerous known faults only to unexpectedly fail at another time. Equipment is lost during a fault clearance on a system that had tested well for ground resistance and was thought to be fully protected.In keeping with the battery analogy, ions are the other critical element in soil conductivity. De-ionized water is, in fact, an effective insulator, but salts in the soil provide the necessary ions to promote current flow. The concentration of salts follows the same pattern as that of moisture percentage. In a typical study done on sandy loam, an increase in percentage of salt by weight of moisture from 0.1 to 1 percent decreased resistivity from 1800 Ω-cm to 460 Ω-cm, while a further increase from 5 to 10 percent decreased it only from 190 Ω-cm to 130 Ω-cm (Table 2). Ion concentration is much more a function of fundamental soil composition than of weather, but weather is not entirely without its effect. Furthermore, that effect might seem counterintuitive. Certain granular types of soil, sand being an example, do not hold ions well, and a concentration of rainfall can actually wash ions away and decrease conductivity. It might be reasonably expected that after a heavy rain, the soil would be saturated with water and a ground test would be expected to read comparatively low. In most instances, this would be true but a surprise may occur, and if it does, make a closer examination of the soil type. Loss of ions is the likely culprit.The other major weather component, of course, is temperature. This does not have as profound an effect as does moisture, but still must be taken into account. T emperature effects can vary in opposite directions on different types of material, so it is wise not to generalize. In soil,decreasing temperature slows the movement of ions and decreases conductivity. Again, the battery analogy holds. The truly critical change occurs over a single degree when going from liquid to ice. If the ground becomes frozen, ice immobilizes the ion flow and resistivity takes a quantum leap. A typical study is once again illustrative. On sandy loam, a drop from 50° to 32° F was found to increase resistivity from 9900 to 13,800 Ω-cm, but in going from liquid to freezing, the same sample increased resistivity to 30,000 Ω-cm! For ground electrode installation, this means that the working structure must be below the frost line, whether that be permafrost or the anticipated worst winter case. Deep-driven rods can ultimately achieve the necessary contact with unfrozen soil, but in areas of shallow bedrock, frost can present a double whammy to the use of multiple shallow rods.Freezing presents another potential problem, and one that is insidious because it is completely unseen. That is, freezing and thawing can exert a mechanical strain on the grounding electrode apart from the electrical stress applied by fault clearance. Grids can separate and sections become lost from connection to the electrical system. The most extreme of weather conditions, lightning, can have a similar effect. Of course, lightning does not have the long-term effect of moisture or temperature, but for the milliseconds that it lasts, it can disintegrate a grounding electrode below grade. The overall effectiveness of what remains can be readily determined by a routine ground test, but high-current grid testers are also available that can indicate damage to the point-to-point structure of the grid itself. This information can be valuable in repairing the grid in order to head off further disintegration.TECH TIPSMoisture Content, y t i v i t s i s e R(Ohm-cm) Percent by Weight Top Soil Sandy Loam0.0 1,000 x 1061,000 x 1062.5 250,000 150,0005.0 165,000 43,00010.0 53,000 22,00015.0 21,000 13,00020.0 12,000 10,00030.0 10,000 8,000*From “An Investigation of Earthing Resistance” by P.J. Higgs, I.E.E. Journal, vol. 68, p. 736, February 1930Table I: Effect of Moisture Content on Earth Resistivity*Added SaltPercent by Weight of Moisture Resistivity, (Ohm-cm)0.0 10,700 0.1 1,800 1.0 460 5.0 190 10.0 13020.0100*For sandy loam; moisture content, 15% by weight; temperature 63º F (17º C)Table II: Effects of Salt Content on Earth Resistivity*W eather averaged out over representative periods produces climate. The one effect to be aware of here is the impact on the water table. This is more likely to be man-mad, by activities like well drilling, but whether man-made or climatic, a change in water table will affect ground resistance. If the water table drops, as by lesser rainfall of the siphoning off from wells, a grounding electrode that was installed in good conductive soil may later be sitting in a much drier environment.What is the effect of all this on ground testing? More than anything, it is important to be aware of the possibilities and not treat ground testing as a once-and-done. The results of a ground resistance test---an installation test, for instance---will be heavily influenced by recent weather conditions. At the time of installation, a once-and-done test could leave the electrical system and associated equipment protected for part of the year only. Around the calendar, the resistance on any given day can vary mightily, and a fault clearance event occurring on the high end of this cycle could result in loss of equipment. Put fundamentally, a ground electrode is only as good as its worst day. In general, grounding conditions are optimal in spring and autumn when weather conditions tend to be moist and reasonably warm. In summer, drought can put the electrical system at risk, and in winter, freezing can present a similar danger. If an installation test is made at an optimal time and just meets spec, there is a high risk of it being considerably out of spec at another time of year. Specialized grounding equipment is availableto mitigate this sawtooth effect by artificially creating a more stable environment around the electrode. This can be accomplished by appropriate backfills, chemical rods, and similar treatments (Fig. 1). But don’t overlook theeffects these treatments may have on concrete foundations, water table, environmental regulations and even the electrode itself. The additional maintenance that may possibly be required must also be taken into account.Knowledge is the most effective tool for field work, and it becomes that much more valuable in applications where variables are as large and uncontrollable as they are in ground testing. Assess the site and recent conditions in order to make an educated decision as to where test results may fall on the min/max cycle, then proceed accordingly. At the least, arrangements should be made to retest at a suspected worst time. If a maintenance schedule is to be established, be judicious about the interval. For most electrical maintenance, a regular schedule, for instance annually or semiannually, is the order of the day, but be careful not to apply this practice to ground maintenance. T esting at regular intervals will result in readings being taken under the same general weather conditions year in and year out. If these are optimal times of year, a false sense of security can develop. Instead, test at irregular intervals such as 5, 7, or 11 months, so that all times of year and all weather conditions will be evaluated. A worst case will be recognized, and, if necessary, the grid can be expanded or improved so that there will be no unpleasant surprises.TECH TIPST esting around the calendar may result in thedisagreeable necessity of working in snow.“When you have to put food on the table in thewintertime, you have to think of these things,”says Burt Brooks of Power Quality & GroundT esting LLC. It may seem counterintuitive, butin frigid climes, snow can provide an advantage.First, the test rods must be driven through thefrost layer. The ground tester must establish aminimum amount of current through the soil inorder to meet its measurement parameters andto sense the voltage drop across the measuredresistance. Modern testers include indicatorsthat will warn the operator if these parametersare not being met. Additional measures mustthen be taken, such as driving deeper rods,to bring the test setup within specifications.Pouring hot water provides only a marginaltemporary advantage and can backfire byfreezing solid around the probe and making itnear impossible to remove. Once an adequate setup is accomplished, Brooks advises, testing under snow is just as reliable as at any other time. Where snow actually can afford an advantage iswhen it falls early in the season before the first major frost. Snow may then insulate the ground and limit frost penetration to more workabledepths, say six to eight inches. If snow has beenplowed or drifted away, frost penetrates deeper and test results may be rendered less consistent. T esting under snow can actually be more reliable.Just shovel away an area large enough to drivethe test rods.The final consideration is that of the testinstrumentation itself. No one is likely to want to perform a ground test in a driving rain, andlightning conditions, even if miles away, are to be avoided because of the risk to the operator. Dangerous voltages developing on the power lines can be transmitted through the grounding system and will appear at the terminals of a tester if a test is in progress. But aside from these extreme circumstances, ground testing can be performed on moist or rainy days, and the sudden appearance of a shower need not send the operator scurrying. The determining factor here is the IP rating of the instrument. This rating should be available in the instrument’s specifications and is commonly referred to asingress protection. It was established by theInternational Electrotechnical Commission(IEC) in Standard #529, and provides a meansof evaluating the effectiveness of an instrument’scasework in keeping out dirt and moisture.The IP rating consists of two numbers, the higher,the better. The first number indicates how wellthe instrument is sealed against particle invasion,with “6” being dust tight. Quarries and minesare particularly bad environments in this regard,while a steady wind in a dusty environmentcan also pose a hazard to the instrument.The second number refers to moisture ingress,with “8” the highest rating representingcontinuous immersion. Since ground testsare not performed under water, this wouldbe overkill, but note the IP rating and obtainan instrument that is adequate to the rigors ofthe field.Armed with knowledge and a good instrument, the skilled technician will be a match for anything the weather can deal.SourceS of information: MEGGER®, A Simple Guide to Earth T esting MEGGER, Getting Down to EarthPower Quality & Ground T esting LLC,Newton, MA Jeffery R. Jowett is a Senior Applications Engineer for Megger in Valley Forge, Pennsylvania, serving the manufacturing lines of Biddle, Megger, and multi-Amp for electrical test and measurement instrumentation. He holds a BS in Biology and Chemistry from Ursinus College. He was employed for 22 years with James G.Biddle Co. which became Biddle Instruments and is now Megger.figure 1: Chemical treatment of soillessens seasonal variation ofelectrodes’ earth resistance。
(完整word)中国农业大学土壤学考博真题答案要点
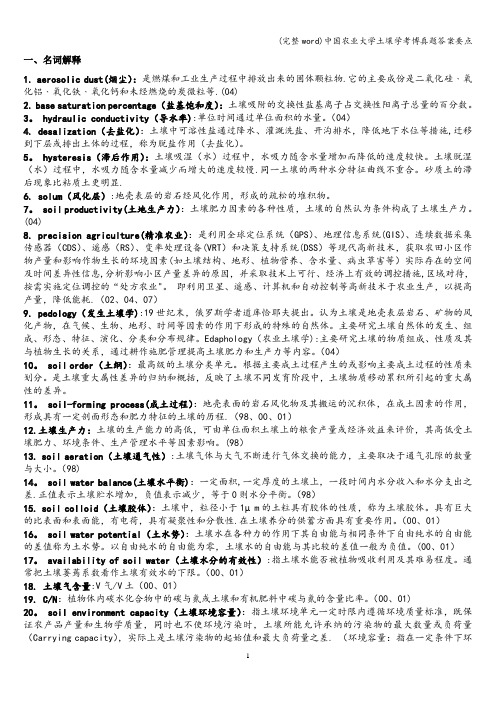
一、名词解释1. aerosolic dust(烟尘):是燃煤和工业生产过程中排放出来的固体颗粒物.它的主要成份是二氧化硅﹑氧化铝﹑氧化铁﹑氧化钙和未经燃烧的炭微粒等.(04)2. base saturation percentage(盐基饱和度):土壤吸附的交换性盐基离子占交换性阳离子总量的百分数。
3。
hydraulic conductivity(导水率):单位时间通过单位面积的水量。
(04)4. desalization(去盐化):土壤中可溶性盐通过降水、灌溉洗盐、开沟排水,降低地下水位等措施,迁移到下层或排出土体的过程,称为脱盐作用(去盐化)。
5。
hysteresis(滞后作用):土壤吸湿(水)过程中,水吸力随含水量增加而降低的速度较快。
土壤脱湿(水)过程中,水吸力随含水量减少而增大的速度较慢.同一土壤的两种水分特征曲线不重合。
砂质土的滞后现象比粘质土更明显.6. solum(风化层):地壳表层的岩石经风化作用,形成的疏松的堆积物。
7。
soil productivity(土地生产力):土壤肥力因素的各种性质,土壤的自然认为条件构成了土壤生产力。
(04)8. precision agriculture(精准农业):是利用全球定位系统(GPS)、地理信息系统(GIS)、连续数据采集传感器(CDS)、遥感(RS)、变率处理设备(VRT)和决策支持系统(DSS)等现代高新技术,获取农田小区作物产量和影响作物生长的环境因素(如土壤结构、地形、植物营养、含水量、病虫草害等)实际存在的空间及时间差异性信息,分析影响小区产量差异的原因,并采取技术上可行、经济上有效的调控措施,区域对待,按需实施定位调控的“处方农业"。
即利用卫星、遥感、计算机和自动控制等高新技术于农业生产,以提高产量,降低能耗.(02、04、07)9. pedology(发生土壤学):19世纪末,俄罗斯学者道库恰耶夫提出。
认为土壤是地壳表层岩石、矿物的风化产物,在气候、生物、地形、时间等因素的作用下形成的特殊的自然体。
-FONT+face=Verdana-水-土相互作用对土体裂隙水流的影响-FONT-
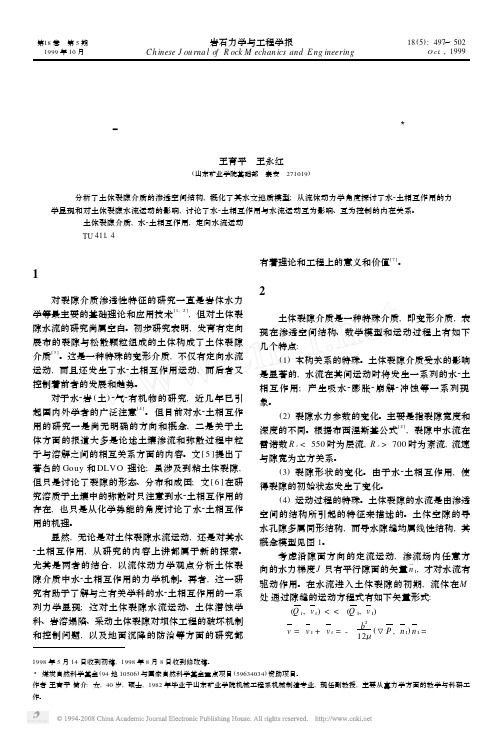
王育平等. 水2土相互作用对土体裂隙水流的影响
·557·
R = F + (P - f )
(8)
式中: F 为水流剪切力, P 为上覆土体的压力, f 为
水对颗粒的浮力。可见剪切力与水力坡度成正比。
当隙中水深变小时, 水力坡度也变小, 这时 F 相应
实验中出现的
5H 5t
临界值是
一个有意义
的现象
(图 5)。当55Ht 大于这一临界值时, 水2土相互作用的 影响处于次要地位; 等于这一值时, 水2土相互作用
和水流运动相互抵消而持平; 低于这一值时, 其作
用就占主导地位。在水流上的表现是流量上升、稳
定和减小。
流量是水2土相互作用与水流运动双方影响的反 应输出。也就是, 流量蕴示着水2土相互作用的程度。 因此, 流量的随机特征, 也说明了水2土相互作用的 过程是一个动态模型。对流量的时序统计分析[3], 进 行在 B IC 准则下的模型识别和估计, 得到其 A R (1) 模型为
·556·
岩石力学与工程学报
1999 年
图 3 含水量与裂隙深度关系图 F ig. 3 R ela tion sh ip of w a ter con ten t and fractu re dep th
图 4 渗流试验的 H 2t, Q 2t (虚线) 曲线 (5. 0 m 隙宽) F ig. 4 Exp erim en ta l cu rves of H 2t and Q 2t (da sh line) w ith w id th of 5. 0 cm
剑桥雅思阅读解析8(test2)
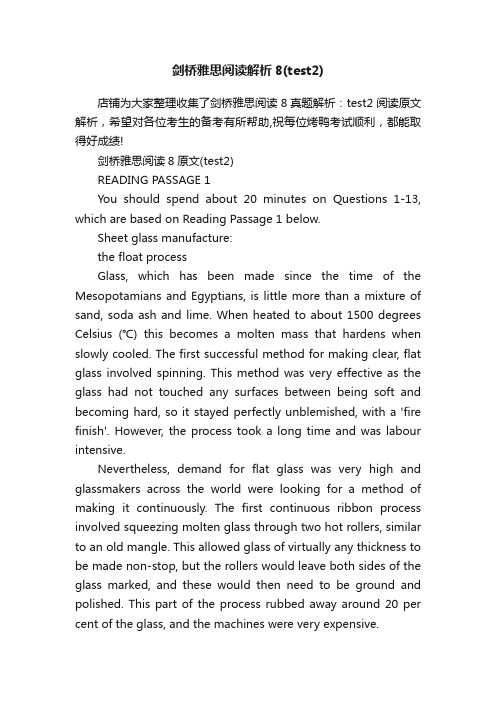
剑桥雅思阅读解析8(test2)店铺为大家整理收集了剑桥雅思阅读8真题解析:test2阅读原文解析,希望对各位考生的备考有所帮助,祝每位烤鸭考试顺利,都能取得好成绩!剑桥雅思阅读8原文(test2)READING PASSAGE 1You should spend about 20 minutes on Questions 1-13, which are based on Reading Passage 1 below.Sheet glass manufacture:the float processGlass, which has been made since the time of the Mesopotamians and Egyptians, is little more than a mixture of sand, soda ash and lime. When heated to about 1500 degrees Celsius (℃) this becomes a molten mass that hardens when slowly cooled. The first successful method for making clear, flat glass involved spinning. This method was very effective as the glass had not touched any surfaces between being soft and becoming hard, so it stayed perfectly unblemished, with a 'fire finish'. However, the process took a long time and was labour intensive.Nevertheless, demand for flat glass was very high and glassmakers across the world were looking for a method of making it continuously. The first continuous ribbon process involved squeezing molten glass through two hot rollers, similar to an old mangle. This allowed glass of virtually any thickness to be made non-stop, but the rollers would leave both sides of the glass marked, and these would then need to be ground and polished. This part of the process rubbed away around 20 per cent of the glass, and the machines were very expensive.The float process for making flat glass was invented by Alistair Pilkington. This process allows the manufacture of clear, tinted and coated glass for buildings, and clear and tinted glass for vehicles. Pilkington had been experimenting with improving the melting process, and in 1952 he had the idea of using a bed of molten metal to form the flat glass, eliminating altogether the need for rollers within the float bath. The metal had to melt at a temperature less than the hardening point of glass (about 600℃), but could not boil at a temperature below the temperature of the molten glass (about 1500℃). The best meta l for the job was tin.The rest of the concept relied on gravity, which guaranteed that the surface of the molten metal was perfectly flat and horizontal. Consequently, when pouring molten glass onto the molten tin, the underside of the glass would also be perfectly flat. If the glass were kept hot enough, it would flow over the molten tin until the top surface was also flat, horizontal and perfectly parallel to the bottom surface. Once the glass cooled to 604℃ or less it was too hard to mark and could be transported out of the cooling zone by rollers. The glass settled to a thickness of six millimetres because of surface tension interactions between the glass and the tin. By fortunate coincidence, 60 per cent of the flat glass market at that time was for six-millimetre glass.Pilkington built a pilot plant in 1953 and by 1955 he had convinced his company to build a full-scale plant. However, it took 14 months of non-stop production, costing the company £100,000 a month, before the plant produced any usable glass. Furthermore, once they succeeded in making marketable flat glass, the machine was turned off for a service to prepare it for years of continuous production. When it started up again it took another four months to get the process right again. They finallysucceeded in 1959 and there are now float plants all over the world, with each able to produce around 1000 tons of glass every day, non-stop for around 15 years.Float plants today make glass of near optical quality. Several processes —melting, refining, homogenising —take place simultaneously in the 2000 tonnes of molten glass in the furnace. They occur in separate zones in a complex glass flow driven by high temperatures. It adds up to a continuous melting process, lasting as long as 50 hours, that delivers glass smoothly and continuously to the float bath, and from there to a coating zone and finally a heat treatment zone, where stresses formed during cooling are relieved.The principle of float glass is unchanged since the 1950s. However, the product has changed dramatically, from a single thickness of 6.8 mm to a range from sub-millimetre to 25 mm, from a ribbon frequently marred by inclusions and bubbles to almost optical perfection. To ensure the highest quality, inspection takes place at every stage. Occasionally, a bubble is not removed during refining, a sand grain refuses to melt, a tremor in the tin puts ripples into the glass ribbon. Automated on-line inspection does two things. Firstly, it reveals process faults upstream that can be corrected. Inspection technology allows more than 100 million measurements a second to be made across the ribbon, locating flaws the unaided eye would be unable to see. Secondly, it enables computers downstream to steer cutters around flaws.Float glass is sold by the square metre, and at the final stage computers translate customer requirements into patterns of cuts designed to minimise waste.Questions 1-8Complete the table and diagram below.Choose NO MORE THAN TWO WORDS from the passage for each answer.Write your answers in boxes 1-8 on your answer sheet.Early methods of producing flat glassMethod Advantages Disadvantages1............Glass remained2........... ? Slow3.............RibbonCould produce glass sheets of varying 4.............non-stop process ? Glass was 5...........20% of glass rubbed awayMachines were expensive图片11Questions 9-13Do the following statements agree with the information given in Reading Passage 1?In boxes 9-13 on your answer sheet, writeTRUE if the statement agrees with the informationFALSE if the statement contradicts the informationNOT GIVEN if there is no information on this9 The metal used in the float process had to have specific properties.10 Pilkington invested some of his own money in his float plant.11 Pilkington’s first full-scale plant was an instant commercial success.12 The process invented by Pilkington has now beenimproved.13 Computers are better than humans at detecting faults in glass.READING PASSAGE 2You should spend about 20 minutes on Questions 14-26, which are based on Reading Passage 2 on the following pages.Question 14-17Reading passage 2 has six paragraphs, A-F.Choose the correct heading for paragraphs B and D-F from the list of headings below.Write the correct number, i-ix, in boxes 14-17 on your answer sheet.List of Headingsi Predicting climatic changesii The relevance of the Little Ice Age todayiii How cities contribute to climate change.iv Human impact on the climatev How past climatic conditions can be determinedvi A growing need for weather recordsvii A study covering a thousand yearsviii People have always responded to climate changeix Enough food at lastExample AnswerParagraph A Viii14 Paragraph BExample AnswerParagraph C V15 Paragraph D16 Paragraph E17 Paragraph FTHE LITTLE ICE AGEA This book will provide a detailed examination of the Little Ice Age and other climatic shifts, but, before I embark on that, let me provide a historical context. We tend to think of climate — as opposed to weather — as something unchanging, yet humanity has been at the mercy of climate change for its entire existence, with at least eight glacial episodes in the past 730,000 years. Our ancestors adapted to the universal but irregular global warming since the end of the last great Ice Age, around 10,000 years ago, with dazzling opportunism. They developed strategies for surviving harsh drought cycles, decades of heavy rainfall or unaccustomed cold; adopted agriculture and stock-raising, which revolutionised human life; and founded the world's first pre-industrial civilisations in Egypt, Mesopotamia and the Americas. But the price of sudden climate change, in famine, disease and suffering, was often high.B The Little Ice Age lasted from roughly 1300 until the middle of the nineteenth century. Only two centuries ago, Europe experienced a cycle of bitterly cold winters; mountain glaciers in the Swiss Alps were the lowest in recorded memory, and pack ice surrounded Iceland for much of the year. The climatic events of the Little Ice Age did more than help shape the modern world. They are the deeply important context for the current unprecedented global warming. The Little Ice Age was far from a deep freeze, however; rather an irregular seesaw of rapid climatic shifts, few lasting more than a quarter-century, driven by complex and still little understood interactions between the atmosphere and the ocean. The seesaw brought cycles of intensely cold winters and easterly winds, then switched abruptly to years of heavy spring and early summer rains, mild winters,and frequent Atlantic storms, or to periods of droughts, light northeasterly winds, and summer heat waves.C Reconstructing the climate changes of the past is extremely difficult, because systematic weather observations began only a few centuries ago, in Europe and North America. Records from India and tropical Africa are even more recent. For the time before records began, we have only 'proxy records' reconstructed largely from tree rings and ice cores, supplemented by a few incomplete written accounts. We now have hundreds of tree-ring records from throughout the northern hemisphere, and many from south of the equator, too, amplified with a growing body of temperature data from ice cores drilled in Antarctica, Greenland, the Peruvian Andes, and other locations, we are close to a knowledge of annual summer and winter temperature variations over much of the northern hemisphere going back 600 years.D This book is a narrative history of climatic shifts during the past ten centuries, and some of the ways in which people in Europe adapted to them. Part One describes the Medieval Warm Period, roughly 900 to 1200. During these three centuries, Norse voyagers from Northern Europe explored northern seas, settled Greenland, and visited North America. It was not a time of uniform warmth, for then, as always since the Great Ice Age, there were constant shifts in rainfall and temperature. Mean European temperatures were about the same as today, perhaps slightly cooler.E It is known that the Little Ice Age cooling began in Greenland and the Arctic in about 1200. As the Arctic ice pack spread southward, Norse voyages to the west were rerouted into the open Atlantic, then ended altogether. Storminess increased in the North Atlantic and North Sea. Colder, much wetter weatherdescended on Europe between 1315 and 1319, when thousands perished in a continent-wide famine. By 1400, the weather had become decidedly more unpredictable and stormier, with sudden shifts and lower temperatures that culminated in the cold decades of the late sixteenth century. Fish were a vital commodity in growing towns and cities, where food supplies were a constant concern. Dried cod and herring were already the staples of the European fish trade, but changes in water temperatures forced fishing fleets to work further offshore. The Basques, Dutch, and English developed the first offshore fishing boats adapted to a colder and stormier Atlantic. A gradual agricultural revolution in northern Europe stemmed from concerns over food supplies at a time of rising populations. The revolution involved intensive commercial farming and the growing of animal fodder on land not previously used for crops. The increased productivity from farmland made some countries self-sufficient in grain and livestock and offered effective protection against famine.F Global temperatures began to rise slowly after 1850, with the beginning of the Modern Warm Period. There was a vast migration from Europe by land-hungry farmers and others, to which the famine caused by the Irish potato blight contributed, to North America, Australia, New Zealand, and southern Africa. Millions of hectares of forest and woodland fell before the newcomers' axes between 1850 and 1890, as intensive European farming methods expanded across the world. The unprecedented land clearance released vast quantities of carbon dioxide into the atmosphere, triggering for the first time humanly caused global warming. Temperatures climbed more rapidly in the twentieth century as the use of fossil fuels proliferated and greenhouse gas levels continued to soar. The rise has been even steeper since theearly 1980s. The Little Ice Age has given way to a new climatic regime, marked by prolonged and steady warming. At the same time, extreme weather events like Category 5 hurricanes are becoming more frequent.Questions 18-22Complete the summary using the list of words, A-I, below.Write the correct letter, A-I, in boxes 18-22 on your answer sheet.Weather during the Little Ice AgeDocumentation of past weather conditions is limited: our main sources of knowledge of conditions in the distant past are 18...........and 19.................. We can deduce that the Little Ice Age was a time of 20.............. , rather than of consistent freezing. Within it there were some periods of very cold winters, other of 21...............and heavy rain, and yet others that saw 22................with no rain at all.A climatic shiftsB ice coresC tree ringsD glaciersE interactionsF weather observationsG heat waves H storms I written accountsQuestions 23-26Classify the following events as occurring during theA Medieval Warm PeriodB Little Ice AgeC Modern Warm PeriodWrite the correct letter, A, B or C, in boxes 23-26 on your answer sheet.23 Many Europeans started farming abroad.24 The cutting down of trees began to affect the climate.25 Europeans discovered other lands.26 Changes took place in fishing patterns.READING PASSAGE 3You should spend about 20 minutes on Questions 27-40, which are based on Reading Passage 3 on the following pages.Questions 27-32Reading Passage 3 has six paragraphs, A-F.Choose the correct heading for each paragraph from the list of headings below.Write the correct number, i-viii, in boxes 27-32 on your answer sheet.List of Headingsi The difficulties of talking about smellsii The role of smell in personal relationshipsiii Future studies into smelliv The relationship between the brain and the nosev The interpretation of smells as a factor in defining groups vi Why our sense of smell is not appreciatedvii Smell is our superior senseviii The relationship between smell and feelings27 paragraph A28 paragraph B29 paragraph C30 paragraph D31 paragraph E32 paragraph FThe meaning and power of smellThe sense of smell, or olfaction, is powerful. Odours affect us on a physical, psychological and social level. For the most part, however, we breathe in the aromas which surround us without being consciously aware of their importance to us. It is only when the faculty of smell is impaired for some reason that we begin torealise the essential role the sense of smell plays in our sense of well-beingA A survey conducted by Anthony Synott at Montreal's Concordia University asked participants to comment on how important smell was to them in their lives. It became apparent that smell can evoke strong emotional responses. A scent associated with a good experience can bring a rush of joy, while a foul odour or one associated with a bad memory may make us grimace with disgust. Respondents to the survey noted that many of their olfactory likes and dislikes were based on emotional associations. Such associations can be powerful enough so that odours that we would generally label unpleasant become agreeable, and those that we would generally consider fragrant become disagreeable for particular individuals. The perception of smell, therefore, consists not only of the sensation of the odours themselves, but of the experiences and emotions associated with them.B Odours are also essential cues in social bonding. One respondent to the survey believed that there is no true emotional bonding without touching and smelling a loved one. In fact, infants recognise the odours of their mothers soon after birth and adults can often identify their children or spouses by scent. In one well-known test, women and men were able to distinguish by smell alone clothing worn by their marriage partners from similar clothing worn by other people. Most of the subjects would probably never have given much thought to odour as a cue for identifying family members before being involved in the test, but as the experiment revealed, even when not consciously considered, smells register.C In spite of its importance to our emotional and sensorylives, smell is probably the most undervalued sense in many cultures. The reason often given for the low regard in which smell is held is that, in comparison with its importance among animals, the human sense of smell is feeble and undeveloped. While it is true that the olfactory powers of humans are nothing like as fine as those possessed by certain animals, they are still remarkably acute. Our noses are able to recognise thousands of smells, and to perceive odours which are present only in extremely small quantities.D Smell, however, is a highly elusive phenomenon. Odours, unlike colours, for instance, cannot be named in many languages because the specific vocabulary simply doesn't exist. ‘It smells like…,’ we have to say when describing an odour, struggling to express our olfactory experience. Nor can odours be recorded: there is no effective way to either capture or store them over time. In the realm of olfaction, we must make do with descriptions and recollections. This has implications for olfactory research.E Most of the research on smell undertaken to date has been of a physical scientific nature. Significant advances have been made in the understanding of the biological and chemical nature of olfaction, but many fundamental questions have yet to be answered. Researchers have still to decide whether smell is one sense or two — one responding to odours proper and the other registering odourless chemicals in the air. Other unanswered questions are whether the nose is the only part of the body affected by odours, and how smells can be measured objectively given the non-physical components. Questions like these mean that interest in the psychology of smell is inevitably set to play an increasingly important role for researchers.F However, smell is not simply a biological and psychologicalphenomenon. Smell is cultural, hence it is a social and historical phenomenon. Odours are invested with cultural values: smells that are considered to be offensive in some cultures may be perfectly acceptable in others. Therefore, our sense of smell is a means of, and model for, interacting with the world. Different smells can provide us with intimate and emotionally charged experiences and the value that we attach to these experiences is interiorised by the members of society in a deeply personal way. Importantly, our commonly held feelings about smells can help distinguish us from other cultures. The study of the cultural history of smell is, therefore, in a very real sense, an investigation into the essence of human culture.Questions 33-36Choose the correct letter, A, B, C or D.Write the correct letter in boxes 33-36 on your answer sheet.33 According to the introduction, we become aware of the importance of smell whenA we discover a new smell.B we experience a powerful smell.C our ability to smell is damaged.D we are surrounded by odours.34 The experiment described in paragraph BA shows how we make use of smell without realising it.B demonstrates that family members have a similar smell.C proves that a sense of smell is learnt.D compares the sense of smell in males and females.35 What is the write doing in paragraph C?A supporting other researchB making a proposalC rejecting a common beliefD describing limitations36 What does the write suggest about the study of smell in the atmosphere in paragraph E?A The measurement of smell is becoming more accurate.B Researchers believe smell is a purely physical reaction.C Most smells are inoffensive.D Smell is yet to be defined.Questions 37-40Complete the sentences below.Choose ONE WORD ONLY from the passage for each answer.Write your answers in boxes 37-40 on your answer sheet.37 Tests have shown that odours can help people recognise the.......... belonging to their husbands and wives.38 Certain linguistic groups may have difficulty describing smell because they lack the appropriate ................ .39 The sense of smell may involve response to................ which do not smell, in addition to obvious odours.40 Odours regarded as unpleasant in certain.................are not regarded as unpleasant in others.剑桥雅思阅读8原文参考译文(test2)PASSAGE 1 参考译文:玻璃板制造:浮法工艺早在美索不达米亚时期和古埃及时期人们就开始制造玻璃,当时制作出的玻璃只不过是沙子、碳酸钠和石灰的混合物而已。
Plant and row spacing effects on soil water and yield of rainfed
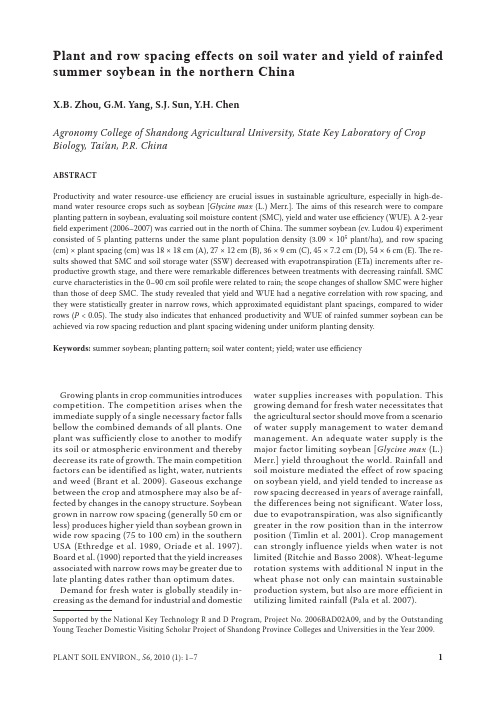
Growing plants in crop communities introduces competition. The competition arises when the immediate supply of a single necessary factor falls bellow the combined demands of all plants. One plant was sufficiently close to another to modify its soil or atmospheric environment and thereby decrease its rate of growth. The main competition factors can be identified as light, water, nutrients and weed (Brant et al. 2009). Gaseous exchange between the crop and atmosphere may also be af-fected by changes in the canopy structure. Soybean grown in narrow row spacing (generally 50 cm or less) produces higher yield than soybean grown in wide row spacing (75 to 100 cm) in the southern USA (Ethredge et al. 1989, Oriade et al. 1997). Board et al. (1990) reported that the yield increases associated with narrow rows may be greater due to late planting dates rather than optimum dates. Demand for fresh water is globally steadily in-creasing as the demand for industrial and domestic water supplies increases with population. This growing demand for fresh water necessitates that the agricultural sector should move from a scenario of water supply management to water demand management. An adequate water supply is the major factor limiting soybean [Glycine max (L.) Merr.] yield throughout the world. Rainfall and soil moisture mediated the effect of row spacing on soybean yield, and yield tended to increase as row spacing decreased in years of average rainfall, the differences being not significant. Water loss, due to evapotranspiration, was also significantly greater in the row position than in the interrow position (Timlin et al. 2001). Crop management can strongly influence yields when water is not limited (Ritchie and Basso 2008). Wheat-legume rotation systems with additional N input in the wheat phase not only can maintain sustainable production system, but also are more efficient in utilizing limited rainfall (Pala et al. 2007).Plant and row spacing effects on soil water and yield of rainfed summer soybean in the northern ChinaX.B. Zhou, G.M. Yang, S.J. Sun, Y.H. ChenAgronomy College of Shandong Agricultural University, State Key Laboratory of Crop Biology, Tai’an, P.R. ChinaABSTRACTProductivity and water resource-use efficiency are crucial issues in sustainable agriculture,especially in high-de-mand water resource crops such as soybean [Glycine max (L.) Merr.]. The aims of this research were to compare planting pattern in soybean, evaluating soil moisture content (SMC), yield and water use efficiency (WUE). A 2-year field experiment(2006–2007) was carried out in the north of China. The summer soybean(cv.Ludou4)experiment consisted of 5 planting patterns under the same plant population density (3.09 × 105plant/ha), and row spacing (cm) × plant spacing (cm) was 18 × 18 cm (A), 27 × 12 cm (B), 36 × 9 cm (C), 45 × 7.2 cm (D), 54 × 6 cm (E). The re-sults showed that SMC and soil storage water (SSW) decreased with evapotranspiration (ETa) increments after re-productive growth stage, and there were remarkable differences between treatments with decreasing rainfall.SMC curve characteristics in the 0–90 cm soil profile were related to rain;the scope changes of shallow SMC were higher than those of deep SMC. The study revealed that yield and WUE had a negative correlation with row spacing,and they were statistically greater in narrow rows, which approximated equidistant plant spacings, compared to wider rows (P < 0.05). The study also indicates that enhanced productivity and WUE of rainfed summer soybean can be achieved via row spacing reduction and plant spacing widening under uniform planting density.Keywords: summer soybean; planting pattern; soil water content; yield; water use efficiencySupported by the National Key Technology R and D Program, Project No. 2006BAD02A09, and by the Outstanding Young Teacher Domestic Visiting Scholar Project of Shandong Province Colleges and Universities in the Year 2009.Previous work on WUE (water use efficiency) primarily dealt with crops grown under water limited conditions and usually did not consider relationship between crop row spacing and WUE (Lehrsch et al. 1994, Graterol et al. 1996, Bowers et al. 2000, Lobato et al. 2009). Increase of WUE is imperative for supplying adequate crop in an environment where future water supplies are ex-pected to decrease. The objective of this study was to derive information on WUE and soil water, which can vary greatly with row spacing of soybean under rainfed agriculture.MATERIALS AND METHODS Experimental design and weather data col-lection. This research was conducted at the Experimental Farm of Shandong Agricultural University, Tai’an (36°09'N, 117°09'E) in northern China. This site is a representative of the main summer soybean growing region of Huanghuaihai Plain in China. The soil samples at the experi-mental site were obtained using a 50 mm diam-eter core from the depths of 0 to 60 cm in each treatment. The soil was a silt loam with the av-erage soil organic matter (SOM) of 16.3 g/kg, N 92.98 mg/kg, P 34.77 mg/kg, K 95.45 mg/kg, and pH of 6.9. Additionally, some physical properties of the soil are given in Table 1. The experiments were established during the growing seasons (from June to September) in 2006 and 2007. As a part of the continuous winter wheat-summer soybean [Glycine max (L.) Merr.] rotation experiment, after winter wheat plants were hand harvested and the stubble removed, summer soybean (cv. Ludou 4) was hand planted on June 12, 2006 and June 13, 2007. The experiment consisted of 5 planting patterns under the same plant population density (3.09 × 105 plant/ha), and row spacing (cm) × plant spacing (cm) was 18 × 18 cm (A), 27 × 12 cm (B), 36 × 9 cm (C), 45 × 7.2 cm (D), 54 × 6 cm (E). Each experiment plot was 3.5 × 6 m in size, and every two plots were separated by a buffer zone 2.0 m wide to reduce mutual effects. Each combination had three replications with a randomized block design. The crops were harvested on September 26, 2006 and September 25, 2007. Other cultural practices were similar to those generally used for non-irrigated summer soybean in the Huanghuaihai Plain, although monthly rainfall data (Table 2) were inadequate to enable water supply from rainfall to be matched with crop ontogeny.Weather data were collected at the Taian Agrometeorological Experimental Station, 500 m from the experimental site. Monthly rain-fall during the summer soybean growing seasons (June–September) is given in Table 2. The long-term average (from 1971 to 2005) rainfall and temperature were 700.5 mm and 12.8°C, and rain-fall was about 520 mm from June to September. The frost-free period was 192 days. Soil water depletion by soybeans was generally confined to soil profile in a depth less than 90 cm (Alessi and Power 1982); therefore, neutron moisture meter access-tubes (one per treatment-replicate) were installed between the rows at each location to a depth of 1.2 m prior to sowing. Soil volumet-ric water content (SWC) was monitored every 10 days throughout the summer soybean grow-ing season at 10-cm depth intervals from 20 to 90 cm using a local field-calibrated CNC503B (DR) Neutron Moisture Probe (Super Energy Nuclear Technology Ltd., Beijing). Water content of the top 20 cm profile of the soil was also determined with portable time domain reflectometry CS620 (TDR) system (Campbell Scientific Australia Pty. Ltd., Townsville), which was used to correct neutron probe data at this depth for all the tubes.Table 1. Some selected physical properties of the experimental siteSoil depth (cm)Bulk density(g/cm3)Field capacity(V. %)Wilting coefficient(V. %)Available water(mm)0–20 1.4836.47.234.8020–40 1.4938.37.536.4440–60 1.5341.28.237.22 Average 1.5038.67.736.15Table 2. Monthly rainfall during 2006–2007 growthseasons of summer soybean (mm)Growth seasonMonthTotal 67892006130.5142.1152.015.3439.9 2007203.4120.4186.029.3539.1Computation and statistical analyses. The ETa for each treatment was computed from planting patterns and climate data from Tai’an Agrometeorological Experimental Station in the area using the following equations:ETa = ∆W + R – SI – Q(1) where: ∆W is change of soil water stored (mm), R is rainfall (mm), SI is deep percolation (mm), Q is surface run-off (mm). SI was estimated using the approach proposed by Gong and Li (1995).SI = ∆W – FK(2) where: FK is field capacity.∆W = ∑(∆Øi × Zi) (3) where: ∆Øi is change in soil volumetric water content (m3/m3) and Zi is depth of the soil layer (mm).Q= (R – 0.2S)2/(R + 0.8S) (4) where: S is potential maximum retention after runoff begins (mm) (Bosznay 1989).S = (25400/CN) –254 (5) where: CN is runoff curve number.WUE = Y/ETa(6) where: Y is grain yield (kg/ha) of summer soybean, ETa is total seasonal evapotranspiration.The statistical significance of different plant and row spacing on yield and water related data was inferred from significance difference tests using analysis of variance accounting for block effects. The least significant difference (LSD, P = 0.05) was used to test for differences in plant-ing patterns (Mishra et al. 2001).RESULTS AND DISCUSSIONThe soil water content (SWC) for different plant and row spacing is shown in Figure 1. Although little difference is shown between treatments within years, there are striking differences between the years themselves. The high SWC average value at the 0–90 cm soil layer in the 2007 growing season might have been affected with 99.2 mm of rain-fall, higher than that in the 2006 growing season; similarly, in 2007, there were similar changes in different soil layers, and the range of SWC fluc-tuations in the upper layer (0–30 cm) was greater compared to deeper layer (60–90 cm) following each row spacing. In the 2006 growing season, there were low SWC values of different soil lay-Figure 1. Dynamic change of soil water content (0–90 cm) under different plant and row spacings of summer soybean in 2006 and 2007 growing seasons. Error bars are standard deviationTime (Y–M–D)ers on June 24; the SWC of treatments decreased with an increase in the soil depth. However, it increased with a decrease in the soil depth since August 14, and reached peak values on July 5, August 4 and September 3. In the 2007 grow-ing season, the high SWC of treatments on June 25 may have been caused by 66.2 mm of rainfall on June 18–23; subsequently, the SWC decreased with decreasing rainfall; the peak values of the SWC in 0–30 cm soil layer on August 3 and September 2 were related to the rainfall of 124.7 and 28.1 mm of from July 14 to August 2 and from 30 to 31 August, respectively.In the 2006–2007 growing seasons, the SWC aver-age value of D treatment was the highest at 0–30 cm soil layer. In low rainfall period, there were evident differences in SWC of different treatments; in the 2006 growing season, the SWC of D treatment was by 20.7% higher than that of C treatment on August 24; in the 2007 growing season, the SWC of D treatment was by 12.8% higher than that of E treatment on July 14. In the 2006 growing season, the maximum differences of the SWC in 30–60 cm and 60–90 cm soil layers appeared on July 5. A treatment was by 25.5% and 68.1% higher than B treatment, respectively; the SWC of 60–90 cm soil layer had a gradually stable trend after August 14. In the 2007 growing season, the SWC of different treatments in 30–90 cm soil layer was similar.The SWC of different growth stages under dif-ferent planting patterns in 2006 and 2007 growing seasons is shown in Figure 2. The results show that in the 2006 growing season, the SWC of different treatments had a ‘Z’ curve trend at the flowering stage (on July 25), pod stage (on August 14) and grain-filling stage (on September 3),and the inflection point of the curve appeared in 40 cm soil layer and 60–80 cm soil layer (Figure 2a). In the same soil lay-ers, the SWC of the flowering stage was lower than that of the pod stage and grain-filling stage.The SWC at the 30–60 cm level was 35–40% in 2007 vs. only 25–30% in 2006, and it appeared that the plants were more drought-stressed in 2006 than in 2007. At different growth stages,there were no evident dif-ferences in the SWC in 0–60 cm soil layer between A treatment and other treatments. At the flowering stage, the SWC of A treatment was the highest in 60–90 cm soil layer. At the pod stage, the SWC of B was by 17.8% lower than that of A treatment in the depth of 90 cm. The SWC of C treatment was me-dium at reproductive phase. At the flowering stage,the SWC of D and E treatments was high in 0–40 cm soil layer, but it decreased in 40–60 cm soil layer; in 70–90 cm soil layer, the SWC of D and E treatments at the grain-filling stage were by 21.4%and 19.4%higher compared to flowering stage,respectively.In the 2007 growing season, the SWC of 10–20 cm soil layer at pod stage (on August 13) and grain-fill-Figure 2. Dynamic changes of soil water in different growth stages under different plant and row spacings in (a)2006 and (b) 2007. Error bars are standard deviation01530456075901015202530354010152025303540101520253035400153045607590Soil water content (%)(a)(b)S o i l d e p t h (c m )Flowering stagePod stageGrain–filling stageing stage (on September 2) was higher than that of the flowering stage (on July 24)(Figure 2b).During whole stages, the order of the SWC average values at the 0–90 cm was A > B ≈ C ≈ D > E, but there were no significant differences recorded (P < 0.05). Especially the SWC profiles during flowering and pod formation stages (the most drought-prone periods for soybean) look very similar across treatments, suggesting that the differences in plant arrangement had little effect on water uptake patterns.Changes of soil storage water (SSW) in summer soybean field during 2006 and 2007 are given in Figure 3. In the 2006–2007 growing seasons, the order of the SSW average values for different treat-ments were D > A > C > E > B, and were 278.6, 278.4, 270.1, 267.4, 266.2 mm (in 2006) and 298.5, 295.3, 293.3, 291.5, 291.2 mm (in 2007), respectively; yet, there were no significant differences (P < 0.05). In the 2006 growing seasons, the SSW showed obvious pre- and post-rainfall changes; before pod stage, the SSW of A treatment was the highest, of C and D treatments were medium, of B and E treatments were lower; from grain-filling stage to mature stage (after August 14), the SSW of D treatment was the highest, of A and E treatments were medium, of B and C treatments were lower. The maximum dif-ference of the SSW appeared on July 5; A treatment was by 20.6% higher than that of B treatment. In the 2007 growing seasons, the SSW of D treatment was comparatively high since July 4, and those of the other treatments showed no evident differences during the stages; the SSW of different treatments showed a descending trend with the advance of the growth stages, which may be relative to less rainfall in September and increased water consumption in the middle and late period of crop growth.Plant and row spacing had obvious effects on yield and WUE in the course of this study on summer soybean (Table 3). There were negligible (and in most cases, non-significant) differences in eva-potranspiration (ETa) among the treatments. Yields in 2007 were about 50% greater than in 2006. In this study, significantly negative correlation between yield and row spacing was observed; the correlation coefficient in 2006 and 2007 (r ) was –0.9257 and –0.9251, respectively (P < 0.05). Yields of A and B treatments were significantly higher than that of E treatment, but no significant differences were recorded among C, D and E treatments (P < 0.05). Yields of the A and B treatments were higher by 36.9% and 35.4% in 2006, and by 19.1% and 21.5% in 2007 than that of E treatment, respectively.Table 3. ETa, yield, WUE, TDM and HI in field under different plant and row spacings of summer soybeanTreatment ETa (mm)Yield (kg/ha)WUE (kg/ha/mm)TDM (kg/ha)HI2006200720062007200620072006200720062007A 312.59a *428.55b 1600a 2615a 5.12a 5.90a 6377a 7756a 0.25a 0.34a B 309.55a 435.29a 1583a 2668a 5.12a 5.92a 5681ab 7752a 0.28a 0.34a C 301.31a 437.16a 1551ab 2340ab 5.15a 5.17ab 6235a 7169ab 0.25a 0.33ab D 319.65a 435.15a 1362ab2265b 4.26b 5.03b 5638ab 7111b 0.24a 0.32ab E306.12a436.07a1169b 2196b3.82c4.87b4837b7504ab0.24a0.29b*values followed by the letter in the same column do not differ significantly using LSD 0.05Figure 3. Changes of soil storage water (0–90 cm) in summer soybean field during whole stages of 2006 and 2007. Error bars are standard deviationS o i l s t o r a g e w a t e r (m m )Time (Y–M–D)There was a significantly negative correlation between WUE and row spacing; the correlation coefficient (r) in 2006 and 2007 was –0.8874 and –0.9382, respectively (P < 0.05). WUE of A and B treatments were significantly higher than those of D and E treatments, respectively (P < 0.05). The total dry matter (TDM) was the highest at A treatment. In the 2007 growing seasons, crop harvest index (HI) of A and B treatments were significantly higher than that of E treatment (P < 0.05). There was a positive correlation between HI and yield, and the correlation coefficient (r) was 0.6288 in 2006 and 0.8434 in 2007 (P < 0.05). The study indicated that enhanced productivity and WUE of rainfed summer soybean can be achieved by reducing row spacing and widening plant spac-ing under uniform planting density.In the 2006 growing season, the SWC values were low at early stage of growth (on June 24) and decreased with an increase in the soil depth; it was affected by 149.8 mm of rainfall during the winter wheat growing seasons (October–June in 2005/2006), out of which 55.2 mm fell between June 1–24, and most of it on June 13–14 then. With less rainfall, the differences in the SWC of shallow (0–30 cm) and deep layer (60–90 cm) were stable. However, similar SWC profiles across treatments within the years indicated that changes in plant arrangement were of no benefit for extracting more water under drought conditions. At the same growing season, the SSW showed a descending trend with ETa increasing after August.Due to annual and seasonal rainfall differences, changes of the SWC curve of different growth stages differed from related reports(Yu et al.2006).In the 2006 growing season, the SWC of the flowering stage was low and the results showed that soil evaporation was the main way of ETa at early stage of growth; the SWC of deep layer was reduced with the increase of crop ETa since pod stage. In the 2007 growing season, the SWC average value of A treatment was high; the results showed that uniform distribution could effectively inhibit soil water evaporation when rainfall was able to meet the water requirements of crop. In the 2006–2007 growing seasons, the SSW of D treatment was comparatively high in the middle and late period of soybean growth, which was prob-ably a result of the effect of plants spacing within rows on reducing soil evaporation; it decreased water requirement of a single plant at late growth stage as inter-plant competition inhibited plant growth at early stage of growth.Increased pod number resulting from greater light interception and crop growth rate during the early reproductive period is mainly responsible for nar-row-row yield increases (Board and Harville 1996). Grain yield increase in response to narrow rows was closely related to the improvement in light in-terception during the critical period for grain set (Andrade et al. 2002). Generally, soybean yield de-clined as clipping timing was delayed and as clipping frequency increased in narrow rows (Singer 2001). The objective of our study was to study the effect of plant and row spacing on soil water and yield of rainfed summer soybean under the same planting density. WUE of D and E treatments were significantly lower than those of A and B treatments, respectively (P < 0.05). It is more likely that the greater WUE of A and B were caused by the narrow-row treatments; they had greater early-season light interception which accelerated crop growth rate resulting in higher yields. Greater yields with equivalent levels of water extraction consequently resulted in greater WUE. The differences in WUE were therefore almost entirely related to differences in seed yield,and it is likely that the observed differences in seed yield were caused by differences in HI(Lawn1982,1983). Thus greater WUE in narrow rows was a symptom. The differences in HI may have reflected treatment differences in the timing of water stress relative to crop ontogeny. The results indicated that D and E treatments showed obvious inter-plant competi-tion. There were significantly negative correlations between WUE and row spacing, and significantly positive correlations between WUE and yield (P < 0.05), which is similar to Ethredge et al. (1989) and Holshouser and Whittaker (2002). The narrow rows (≤ 40 cm) should be used to optimize yields from the Early Soybean Production System plantings in the midsouthern USA (Bowers et al. 2000). The study found that seed yield and WUE were statistically greater in narrow rows (row spacing ≤ 27 cm), with approximate equidistant plant spacing, compared to wider rows (row spacing ≥ 36 cm).The study over 2 years has shown that high yields and WUE of summer soybean can be achieved in the northern China by reducing row spacing and widening plant spacing under uniform planting density. Similar SWC values across treatments within years indicate that changes in plant ar-rangement of summer soybean are of no benefit for extracting more water under rainfed agriculture. During whole stages, the SWC average value at the 0–90 cm had no significant differences (P < 0.05), suggesting that differences in plant arrangement have little effect on water uptake patterns. The conclusion of the study is that row spacing ≤ 27 cm is high optimum.REFERENCESAlessi J., Power J.F. (1982): Effects of plant and row spacing on dryland soybean yield and water-use efficiency. Agronomy Journal, 74: 851–854.Andrade F.H., Calvino P., Cirilo A., Barbieri P. (2002): Yield re-sponses to narrow rows depend on increased radiation inter-ception. Agronomy Journal, 94: 975–980.Board J.E., Harville B.G. (1996): Growth dynamics during the vegetative period affects yield of narrow-row, late-planted soybean. Agronomy Journal, 88: 567–572.Board J.E., Harville B.G., Saxton A.M. (1990): Narrow-row seed-yield enhancement in determinate soybean. Agronomy Journal, 82: 64–68.Bosznay M. (1989): Generalization of SCS curve number method. Journal of Irrigation and Drainage Engineering, 155: 139–144.Bowers G.R., Rabb J.L., Ashlock L.O., Santin J.B. (2000): Row spacing in the early soybean production system. Agronomy Journal, 92: 524–531.Brant V., Neckář K., Pivec J., Duchoslav M., Holec J., Fuksa P., Venclová V. (2009): Competition of some summer catch crops and volunteer cereals in the areas with limited precipitation. Plant, Soil and Environment, 55: 17–24.Ethredge W.J., Ashley D.A., Woodruff J.M. (1989): Row spac-ing and plant population on yield components of soybean. Agronomy Journal, 81: 947–951.Gong Y.S., Li B.G. (1995): Using field water balance model to estimate the percolation of soil water. Advanced Water Sci-ences, 6: 16–21. (In Chinese)Graterol Y.E., Elmore R.W., Eisenhauer D.E. (1996): Narrow-row planting systems for furrow-irrigated soybean. Journal of Pro-duction Agriculture, 9: 546–553.Holshouser D.L., Whittaker J.P. (2002): Plant population and row-spacing effects on early soybean production systems in the Mid-Atlantic USA. Agronomy Journal, 94: 603–611. Lawn R.J. (1982): Response of four grain legumes to water stress in south eastern Queensland III: Dry matter production, yield and water use efficiency. Australian Journal of Agricultural Research, 33: 511–wn R.J. (1983): Response of four grain legumes to water stress in south east Queensland IV. Interaction with sowing arrangement. Australian Journal of Agricultural Research, 34: 661–669. Lehrsch G.A., Whisler F.D., Buehring N.W. (1994): Cropping system influences on extractable water for mono-and dou-ble-cropped soybean. Agricultural Water Management, 26: 13–25.Lobato A.K.S., Costa R.C.L., Oliveira Neto C.F., Santos Filho B.G., Gonçalves-Vidigal M.C., Vidigal Filho P.S., Silva C.R., Cruz F.J.R., Carvalho P.M.P., Santos P.C.M., Gonela A. (2009): Consequences of the water deficit on water relations and sym-biosis in Vigna unguiculata cultivars. Plant, Soil and Environ-ment, 55: 139–145.Mishra H.S., Rathore T.R., Savita U.S. (2001): Water-use efficiency of irrigated winter maize under cool weather conditions of India. Irrigation Science, 21: 27–33.Oriade C.A., Dillon C.R., Vories E.D., Bohanan M.E. (1997): An economic analysis of alternative cropping and row spacing systems for soybean production. Journal of Production Agri-culture, 10: 619–624.Pala M., Ryan J., Zhang H., Singh M., Harris H.C. (2007): Water-use efficiency of wheat-based rotation systems in a Mediter-ranean environment. Agricultural Water Management, 93: 136–144.Ritchie J.T., Basso B. (2008): Water use efficiency is not con-stant when crop water supply is adequate or fixed: the role of agronomic management. European Journal of Agronomy, 28: 273–281.Singer J.W. (2001): Soybean light interception and yield response to row spacing and biomass removal. Crop Science, 41: 424–429.Timlin D., Pachepsky Y., Reddy V.R. (2001): Soil water dynamics in row and interrow positions in soybean (Glycine max L.). Plant and Soil, 237: 25–35.Yu S.Z., Chen Y.H., Li Q.Q., Zhou X.B., Fang Q.X., Wang J.S., Liu E.M., Luo Y. (2006): Feasible study on water-saving effect of wheat-maize rotation pattern. Acta Ecology Sinica, 26: 2523–2531. (In Chinese)Received on April 5, 2009Corresponding author:Dr. Yu Hai Chen, Agronomy College of Shandong Agricultural University, State Key Laboratory of Crop Biology, 271018 Tai’an, P.R. Chinae-mail: yhchen@。
Effects of water
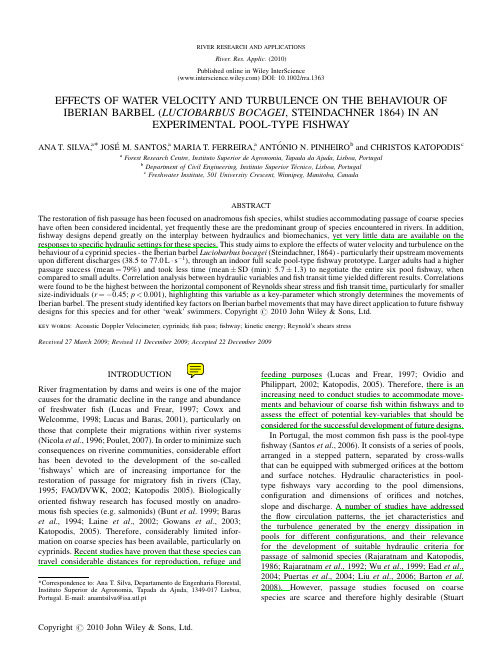
EFFECTS OF WATER VELOCITY AND TURBULENCE ON THE BEHA VIOUR OF IBERIAN BARBEL(LUCIOBARBUS BOCAGEI,STEINDACHNER1864)IN ANEXPERIMENTAL POOL-TYPE FISHWAYANA T.SILV A,a*JOSE´M.SANTOS,a MARIA T.FERREIRA,a ANTO´NIO N.PINHEIRO b and CHRISTOS KATOPODIS ca Forest Research Centre,Instituto Superior de Agronomia,Tapada da Ajuda,Lisboa,Portugalb Department of Civil Engineering,Instituto Superior Te´cnico,Lisboa,Portugalc Freshwater Institute,501University Crescent,Winnipeg,Manitoba,CanadaABSTRACTThe restoration offish passage has been focused on anadromousfish species,whilst studies accommodating passage of coarse species have often been considered incidental,yet frequently these are the predominant group of species encountered in rivers.In addition,fishway designs depend greatly on the interplay between hydraulics and biomechanics,yet very little data are available on the responses to specific hydraulic settings for these species.This study aims to explore the effects of water velocity and turbulence on the behaviour of a cyprinid species-the Iberian barbel Luciobarbus bocagei(Steindachner,1864)-particularly their upstream movements upon different discharges(38.5to77.0LÁsÀ1),through an indoor full scale pool-typefishway rger adults had a higher passage success(mean¼79%)and took less time(meanÆSD(min):5.7Æ1.3)to negotiate the entire six poolfishway,when compared to small adults.Correlation analysis between hydraulic variables andfish transit time yielded different results.Correlations were found to be the highest between the horizontal component of Reynolds shear stress andfish transit time,particularly for smaller size-individuals(r¼À0.45;p<0.001),highlighting this variable as a key-parameter which strongly determines the movements of Iberian barbel.The present study identified key factors on Iberian barbel movements that may have direct application to futurefishway designs for this species and for other‘weak’swimmers.Copyright#2010John Wiley&Sons,Ltd.key words:Acoustic Doppler Velocimeter;cyprinids;fish pass;fishway;kinetic energy;Reynold’s shears stressReceived27March2009;Revised11December2009;Accepted22December2009INTRODUCTIONRiver fragmentation by dams and weirs is one of the major causes for the dramatic decline in the range and abundance of freshwaterfish(Lucas and Frear,1997;Cowx and Welcomme,1998;Lucas and Baras,2001),particularly on those that complete their migrations within river systems (Nicola et al.,1996;Poulet,2007).In order to minimize such consequences on riverine communities,considerable effort has been devoted to the development of the so-called ‘fishways’which are of increasing importance for the restoration of passage for migratoryfish in rivers(Clay, 1995;FAO/DVWK,2002;Katopodis2005).Biologically orientedfishway research has focused mostly on anadro-mousfish species(e.g.salmonids)(Bunt et al.1999;Baras et al.,1994;Laine et al.,2002;Gowans et al.,2003; Katopodis,2005).Therefore,considerably limited infor-mation on coarse species has been available,particularly on cyprinids.Recent studies have proven that these species can travel considerable distances for reproduction,refuge and feeding purposes(Lucas and Frear,1997;Ovidio and Philippart,2002;Katopodis,2005).Therefore,there is an increasing need to conduct studies to accommodate move-ments and behaviour of coarsefish withinfishways and to assess the effect of potential key-variables that should be considered for the successful development of future designs. In Portugal,the most commonfish pass is the pool-type fishway(Santos et al.,2006).It consists of a series of pools, arranged in a stepped pattern,separated by cross-walls that can be equipped with submerged orifices at the bottom and surface notches.Hydraulic characteristics in pool-typefishways vary according to the pool dimensions, configuration and dimensions of orifices and notches, slope and discharge.A number of studies have addressed theflow circulation patterns,the jet characteristics and the turbulence generated by the energy dissipation in pools for different configurations,and their relevance for the development of suitable hydraulic criteria for passage of salmonid species(Rajaratnam and Katopodis, 1986;Rajaratnam et al.,1992;Wu et al.,1999;Ead et al., 2004;Puertas et al.,2004;Liu et al.,2006;Barton et al. 2008).However,passage studies focused on coarse species are scarce and therefore highly desirable(StuartRIVER RESEARCH AND APPLICATIONSRiver.Res.Applic.(2010)Published online in Wiley InterScience()DOI:10.1002/rra.1363 *Correspondence to:Ana T.Silva,Departamento de Engenharia Florestal,Instituto Superior de Agronomia,Tapada da Ajuda,1349-017Lisboa,Portugal.E-mail:anamtsilva@isa.utl.ptand Mallen-Cooper,1999;Katopodis,2005).This require-ment is highlighted in Iberian rivers where cyprinidfishes are frequently the most abundant group of species encountered(Doadrio,2001).The Iberian barbel Luciobarbus bocagei(Steindachner, 1864)is one of the most common cyprinid species in Iberian rivers,though its migration ecology has been assessed only in recent studies(Santos et al.,2005).However,little is known about this species’response to specific hydraulic characteristics,such as water velocity and turbulence.These two hydraulic variables are considered to play an essential role in successfulfish passage through afishway(Rajar-atnam et al.,1998).Turbulent kinetic energy(K)and Reynolds shear stress are two interdependent turbulence descriptors that naturally affectfish migration,but for which, detailed knowledge on their effects onfish movements is scarce(Rajaratnam et al.,1988;Odeh et al.,2002).Due to the fact that both turbulence kinetic energy and Reynolds shear stress are important indicators of turbulence effect on a fish’s body,it is clear there is a need to understand how Iberian barbel sense,react and use these hydraulic phenomena in their movements withinfishways,in order to develop robust guidelines that are useful for future fishway designs.However,their assessment under natural conditions is extremely difficult and possibly inaccurate due to the plethora of interacting factors.To be successful studies should be based on balanced experimental designs in which the variables of interest can be manipulated.Studies conducted on such conditions offer an excellent opportunity to gain generic insights intofish behaviour(Kemp et al., 2006).The main goal of this study is to understand the effects of water velocity and turbulence,expressed as turbulent kinetic energy(K)and Reynolds shear stress,on the upstream movements of Iberian barbel under experimental conditions in a full scale pool-typefishway.Specifically,the following questions should be answered:(a)how do the different hydraulic parameters relate to the transit time of distinctfish size-classes within thefishway,i.e.,are smaller size-classes more affected by hydraulics than larger conspecifics?and(b) which hydraulic parameters affectfish performance within thefishway the most?MATERIALS AND METHODS ExperimentalfishwayThe study was conducted in an indoor full scale pool-typefishway installed at the Hydraulics and Environment Department of the National Laboratory for Civil Engineer-ing(LNEC),in Lisbon.The structure was10m long,1m wide and1.2m high,externally reinforced byfiberglass sidewalls(Figure1).It consisted of six pools(1.9m longÂ1.0m wideÂ1.2m high)divided byfive PVC cross-walls,each equipped with a submerged orifice and a surface notch of adjustable area.Two consecutive orifices were positioned on opposite sides of the cross-walls,creating a sinusoidalflow path.Thefishway was set at a slope of8.5%, which is within the range of slopes commonly used in this type of hydraulic devices(Larinier,2002).A concrete tank (1.5mÂ1.0mÂ1.2m)located at the upstream end of the flume,provided a smoothflow entering in theflume. Thefishway also encompassed an acclimation chamber (4.0mÂ3.0mÂ4.0m)at the downstream end of the channel,which was separated from the main structure by two mesh panels.Water used in the experiments was drawn from domestic water supply(soft water)and was recirculated through the laboratory pumping system (maximum capacity of259LÁsÀ1)for at least2weeks prior to the experiments to ensure dechlorination and ‘maturation’throughout(Copp et al.,1998),and checked for temperature,hardness,pH and dissolved oxygen at the beginning and at the end of experiments by means of a multiparametric probe(Hydrolab,Quanta model). Fishway discharge was measured by a magneticflow meter located in the supply pipe and controlled by a slot valve at the upstream tank.Water surface levels were measured using graduated scales placed on the side-wall of the pool,in the vicinity of the cross-walls and middle point of the pool.The water level within the structure was regulated by a slot gate at the downstream end of the facility.77.0L sÀ1,and the area of submerged orifices(A o)between 0.03and0.06m2,four experimental conditions were tested (Table I).Because Silva et al.(2009)found that Iberian barbel avoided notched surface openings,the surface notches,under all experiments,remained closed. Detailed instantaneous velocity measurements were conducted using an instantaneous3D Acoustic Doppler Velocimeter(ADV)oriented vertically down(Nortek AS). The advantage of using this device relies on its ability to adequately measure the three-dimensional velocity com-ponents(x,y,z)offlowing water(Papanicolaou and Maxwell,2000;Odeh at al.,2002;Guiny et al.,2003;Ead et al.,2004).Velocity measurements were recorded at 25Hz for a sampling period of90s in each point on the grid.Preliminary tests were conducted to define the ADV sampling period needed for an accurate determination of the mean velocity,turbulent kinetic energy and Reynolds shear stress.Tested sampling periods ranged from5to350s. It was found that velocity became almost constant for sampling periods greater than30s,therefore a samplingA.T.SILV A ET AL.time of 90s was considered to be representative for an appropriate determination of mean velocity and turbulence within the pool.Because the flow pattern and the head drop between the pools were found to be the same in all pools (D h ¼0.16m),measurements were made in the second downstream pool.Measurements were taken at different horizontal planes parallel to the flume bottom,namely at 25,50and 80%of the pool mean depth (h m ).A predefined grid of 48measurement points distributed according to the velocity fluctuations expected was used as a reference to the measurements in each plane.Altogether,2500instantaneous measurements were recorded for each sampling point.To understand the velocity fields at the different planes –horizontal (XY),vertical (XZ)and transversal (YZ)–withinTable I.Details of experimental conditions:submerged orifices area (A o ),head drop between pools (D h ),flow discharge (Q),volumetric power dissipation (P v ),pool mean water depth at 25,50and 80%.Fish size of individuals used in the experiments are also shown ExperimentsVariables A o (m 2)D h (m)Q (L Ás À1)P v(W Ám À3)h m (m)25%h m (m)50%h m (m)80%h m (m)Small adults:15<TL <25cm Large adults:25 TL <35cm NMean ÆS.D(cm)N Mean ÆS.D(cm)E10.030.1638.537.00.790.200.400.631019.07Æ1.761028.87Æ2.59E20.040.1647.547.80.860.210.430.681019.85Æ2.491028.44Æ3.11E30.050.1662.763.10.850.210.420.681019.74Æ2.141028.67Æ2.89E40.060.1677.078.40.840.210.420.671019.65Æ2.411028.25Æ3.07Figure 1.(a)Side view of the experimental full scale pool-type fishway at the National Laboratory for Civil Engineering;(b)reference grid used for fishbehaviour observationIBERIAN BARBEL’S RESPONSE TO VELOCITY AND TURBULENCEthe pools,the ratios between the maximum velocity in each plane(Vxy m,Vxz m and Vyz m)and the maximumflow velocity at the orifice(V o)were calculated for the four experiments.Turbulence descriptors–turbulent kinetic energy and Reynolds shear stress–were calculated based on the instantaneous velocities registered.The local velocity in a turbulent region can be decomposed of a temporal mean value plus a component that represents the velocity fluctuation about the mean.Accordingly:VðtÞ¼VþV0ðtÞ(1) where V is the mean velocity at the point during the sampling period and V0(t)is thefluctuating component of velocity at sampling time t.Turbulent kinetic energy(K)is defined as:K¼12u02rmsþv02rmsþw02rmsÀÁ(2)where u0,v0and w0are the longitudinal,transversal and verticalfluctuating velocities,respectively.This parameter represents the kinetic energy of the velocityfluctuations,i.e. greaterfluctuation from the meanflow represents greater turbulent kinetic energy.The size of the turbulent fluctuations is therefore important to consider when studying upstream movements offish species.Small-scale turbulence associated with tiny eddies may cause localized damage on fish body surfaces(bruises,scale loss),whereas larger-scale turbulence,i.e.greater thanfish size,can cause spin,fish disorientation and loss of equilibrium which ultimately affect their upstream movement(Odeh et al.,2002).To enable comparisons with others studies,K was formulated in dimensionless terms(normalized)using the maximum velocity in the orifice(V o),according to the following equation:k¼K0:5=V o(3) The Reynolds shear stress represents anotherfluid force that involves a velocity gradient,and therefore is of major importance forfish passage studies.This parameter represents a force per unit area and occurs when two water masses or layers of different velocities are parallel or adjacent to each other.Fish may therefore experience shear stress when moving between two water masses of different velocities or when moving near a solid structure (Cada et al.,1999).To better understand which forces are primarily acting on the body surface offish and therefore influencing their upstream movements,this parameter was partitioned on three components according to the forces present on each plane:longitudinal(forces acting on the XY plane),vertical (forces acting on the XZ plane)and transversal(forces acting on the YZ plane).For the horizontal component,shear stress is defined by:Àr u0v0;(4) where r is the water density,u0and v0are thefluctuating velocities in the X and Y directions,respectively.The variations of the Reynolds shear stress in each of the three dimensions were therefore assessed at two different layers from theflume bottom:at0.25h m(close to the bottom) and at0.80h m(near the surface).To allow for comparisons, this parameter was also made dimensionless according to the maximum velocity in the orifice(V o)according to the following equation,for the horizontal plane(XY):ðÀu0v0Þm.V2o(5)where the subscript m refers the maximum value in a transverse profile.The variations of the dimensionless Reynolds shear stress at the verticalðÀu0w0Þm.V2oand at the transversal planeðÀv0w0Þm.V2owere also calculated to z¼0.25h m and z¼0.80h m.FishIberian barbels were capturedat the River Sorraia,the largest tributary of the River Tagus(central Portugal). Further details of the sampling area are included in Collares-Pereira et al.(1995).Sampling was undertaken using electrofishing(Electrocatch International,Sarel model WFC7_HV,Wolverhampton,UK)with low voltage (250v)and with a30cm diameter anode.Fish were captured during the natural reproductive migration season, which generally takes place from April to June(Santos et al., 2005).Only mature individuals,as shown by in situ macroscopic observations offish gonads development stage, particularly their volume,vascular irrigation and visibility of oocytes,and the presence of nuptial tubercles(Agostinho et al.,2007)were selected for the study.Fish(N¼80)were brought to the laboratory,and measured for total length (TL).To test for size-related differences in species behaviour,fish were separated into two size-classes according to reported differences in age and growth(Lobo´n-Cervia´, 1982;Lobo´n-Cervia´and Ferna´ndez-Delgado,1984), although such divisions are somewhat arbitrary and flexible depending on catchment characteristics(Oliveira et al.,2002):small(15<TL<25cm)and large adults (25TL<35cm).Iberian barbel of each size-class(n¼40; 20individuals per tank)were then placed in four tanks(lengthÂwidthÂheight¼1.45mÂ0.70mÂ0.80m) equipped with ELITE aerator systems and mechanical filters.To recover from transport and handling stress,fish were kept in the tanks for at least1week before experiments started.During that period,fish were fedA.T.SILV A ET AL.daily with pond sticks (Tetra Pond),until 24h prior to experimentation.The difference in water temperature between the tanks and the fishway (mean temperature ¼188C)was less than 28C.Fish experiments took place between the 10th and 18th of May 2007from 17h00to 22h30,to encourage attempts by this species which typically migrates at dusk (Santos et al .,2005).Each experiment lasted 1.5h,as a previous pilot study (unpublished data)–employing the same design settings and holding conditions,but where barbel were left for 5h (17h00-22h00),from dusk to early-night,to ascend the fishway have shown no further fish movements into the flume after the above time period.Experiments were conducted by using one adult fish of each size-class simultaneously.Individuals were first introduced in the acclimation chamber where they remained for 12h prior to experimentation and were prevented from entering the flume by two mesh panels.Once the discharge in the flume was brought to the desired level,the mesh panels were removed and fish were allowed to ascend the fishway of their own volition.Fish experiments were conducted under the four previously tested hydraulic conditions with 10replicates each,giving a total of 40trials.Fish behaviour was continuously monitored through the glass sidewalls of the fishway by means of direct observation and a video recording system.Direct observations were performed during the entire time of an experiment and were made at and leaving discreetly the observation points,respectively before and after each experiment.This caused no disturbance to the fish,as revealed by a previous study (Silva et al.,2009).Each operator followed a single fish,registering continuously all of its movements after removal of both mesh panels,including the time taken by the fish (i)to enter the flume,(ii)to ascend from one pool to the next and (iii)to successfully negotiate the fishway.Observations were also supplemented by three digital video cameras focused on the second pool,which was considered to be representative of the remaining hydraulic conditions (see Hydraulics).Two of the cameras were positioned at a distance of 2m from both side-walls of the pool,whereas the other was placed 3m above the water surface.In addition,a 1.90m Â1.00m reference grid containing 15contiguous sequentially numbered cells (each 0.38m Â0.33m)were created and placed above this pool to aid in the video monitoring process (Figure 1).Video records were analyzed by a single operator,using the IVision Labview software from National Instruments (),allowing the collection of continuous exact locations of fish within the pool.An individual fish was considered to occupy one cell,when more than half of its body length was within a cell’s boundaries.The time spent by a fish in each cell of the grid (transit time)wasdetermined and related to the hydraulic parameters values previously found.Statistical analysisKruskal–Wallis ANOV A was employed to test the hypothesis that,for each fish size-class,the transit times in each cell of the grid were equivalent among experiments.Correlations between transit time and mean velocity,turbulent kinetic energy and three-dimensional Reynolds shear stresses were analysed using the Spearman rank coefficient.Data were pooled over the four experimental conditions,as a previous analysis revealed water velocity vectors to be spatially similar among experiments,though increasing proportionally in terms of magnitude with increasing flow discharge.Analyses were performed using data collected at z ¼0.25h m instead of considering mean values obtained from all three planes,as visual observations and video monitoring showed that fish movements were found to occur preferentially close to the bottom of the fishway.RESULTSHydraulicsThe mean velocity patterns for the three horizontal planes at 0.25h m ,0.50h m and 0.80h m above the bottom of the flume for Q ¼47.5L Ás À1are shown in Figure 2.In the horizontal plane at 0.25h m ,closest from the flume bed,two different regions could be clearly distinguished:the jet region,with maximum velocities occurring at a distance of 0.40m downstream from the cross-wall and reaching 0.83m Ás À1,and a large recirculation region,extending from the jet zone to the opposite side-wall,characterized by low velocities and reversed flow directions.At the plane closest to the water surface (0.80h m ),a uniform recircula-tion region could be observed,with moderate to high velocities (0.50–0.77m Ás À1),occurring along this plane,particularly in the vicinity of the downstream cross-wall.The ratio between the maximum velocity in each plane (Vxy m ,Vxz m and Vyz m )and the maximum velocity in the orifice (V o )are shown in Figure 3.It can be seen that in the horizontal plane (XY),maximum velocities occur near the bottom of the flume increasing with flow discharge (Figure 3a).In both vertical (XZ)and transversal (YZ)planes,the occurrence of higher velocities with increasing discharge was less evident.Nonetheless,patterns of velocity distribution could still be observed along the pool,particularly at higher discharges:on a vertical plane,maximum velocities were found to be greater in the vicinity of the side-wall adjacent to the orifice and decreasing towards the opposite side-wall (Figure 3b).Similarly on aIBERIAN BARBEL’S RESPONSE TO VELOCITY AND TURBULENCEFigure 2.Plane velocity field in the pools for Q ¼47.5L Ás À1:(a)z ¼0.25h m ;(b)z ¼0.50h m ;(c)z ¼0.80h m .Flow from the orifice enters at the bottom left ofthe diagramA.T.SILV A ET AL.transverse plane,the maximum velocities varied slightly with the increasing discharge peaking in the vicinity of both cross-walls (0.40V o ),ranging between 0.20and 0.30V o in the remaining areas (Figure 3c).The contours of the dimensionless turbulent kinetic energy (Q ¼47.5L Ás À1)at z ¼0.25h m,and z ¼0.80h m are shown in Figure 4.Turbulence was found to be higher near the bottom of the flume (z ¼0.25h m ),particularly along the streamline between the submerged orifices.At the level closest to the surface (z ¼0.80h m ),a rapid decay was observed within the same jet region.In contrast,in the recirculation area,turbulence remained low (around 0.08–0.10V o )at both levels,though a small increase was noted near the surface.The variations of Reynolds shear stress in all three planes are shown in Figure 5.The absolute value of this parameter was found to decrease in both the horizontal and vertical planes when moving from the bottom (z ¼0.25h m )to thesurface (z ¼0.80h m ).Accordingly,at z ¼0.25h m ,the values of absolute horizontal (Figure 5a)and vertical shear stress (Figure 5c)were greater in the vicinity of the upstream cross-wall (flow inlet),decreasing from the middle part of the pool towards the downstream end,with these patterns being clearer at higher discharges.At the level near the surface (z ¼0.80h m ),absolute shear forces remained almost constant along the pool length and among experiments,ranging from 0to 0.01(Figure 5b and Figure 5d,respectively).Variations in transverse Reynolds shear stress at z ¼0.25h m (Figure 5e)and z ¼0.80h m (Figure 5f)were quite low,remaining almost constant along the pool length and throughout experiments.FishIn all the experiments,fish exhibited a high capacity to negotiate the fishway,though some size-related differences were noted (Figure 6a).Accordingly,larger adults presented a higher rate of success (mean ¼79%)in ascending the fishway,relative to small adults (mean ¼59%).Similarly,larger adults took less time (mean ÆSD (min):5.7Æ1.3)to ascend the fishway,when compared to small-size individuals (mean ÆSD (min):8.0Æ0.4)(Figure 6b).Transit time did not differ among experiments (Kruskal–Wallis ANOV A:p >0.05)and was found to be lower withFigure 3.Dimensionless maximum velocities at various tested discharges on different planes:(a)horizontal plane (XY );(b)vertical plane (XZ );(c)transversal plane (YZ )Figure 4.Contours of dimensionless turbulent kinetic energy k ¼K 0:5V o ,for Q ¼47.5L Ás À1at different horizontal planes:(a)z ¼0.25h m ;(b)z ¼0.80h m .Flow from the orifice enters at the bottom left of the diagramIBERIAN BARBEL’S RESPONSE TO VELOCITY AND TURBULENCEincreasing mean velocity for both size-classes,particularly for small adults (Spearman rank correlation:small adults:r ¼À0.30,p <0.05;large adults:r ¼À0.27,p <0.05).The relationship between mean values of velocity within eachcell (see Figure 1b for a general scheme)and the respective fish transit time at z ¼0.25h m is shown in Figure 7.Both size-classes used cells with low velocity values (0.20–0.40m Ás À1).Figure 5.Variation of dimensionless Reynolds shear stress at various tested discharges on different planes:(a)horizontal plane (XY )at z ¼0.25h m (b)horizontal plane (XY )at z ¼0.80h m (c)vertical plane (XZ )at z ¼0.25h m (d)vertical plane (XZ )at z ¼0.80h m (e)transversal plane (YZ )at z ¼0.25h m (f)transversal plane (YZ )at z ¼0.80h m .Here,flow enters from the right of the diagramA.T.SILV A ETAL.The plot of fish transit time with turbulent kinetic energy (K)is shown in Figure 8for the different cells within the pool.It shows that small (Figure 8a)and large adults (Figure 8b)used mainly areas with low K (<0.05m 2Ás À2).Therefore,a significant negative correlation between K and fish transit time was found for both fish sizes (Spearman rank correlation:small adults:r ¼À0.39,p <0.01;large adults:r ¼À0.35,p <0.01).Finally,the relation between Reynolds shear stress components (horizontal,vertical and transversal)and fish transit time in each cell is shown in Figure 9.Correlations between the former and the components of Reynolds shear stress in the different planes yielded different results.Accordingly,a negative association between fish transit time and the horizontal shear stress was found for both size-classes,particularly for small adults (Figure 9a and Figure 9b)(Spearman rank correlation:small adults:r ¼À0.45,p <0.001;large adults:r ¼À0.36,p <0.01).Overall,individuals spent less time in cells with higher shear stress values (absolute values ranged from 20to 60N Ám À2)on this plane.This correlation was the strongest among all tested hydraulic characteristics against transit time,thereby indicating that the horizontal componentofFigure 6.(a)Percentage of fish that successfully negotiated the entire six pool fishway;(b)time taken by fish to negotiate the entire six pool fishway:small adults (15<TL <25cm)(&)and large adults (25 TL <35cm)(&)Figure 7.Distribution of transit time per cell(s)versus mean velocity in each cell (U c )at z ¼0.25h m for various tested discharges:(a)small adults(15<TL <25cm)and (b)large adults (25 TL <35cm)Figure 8.Distribution of transit time per cell(s)versus mean turbulent kinetic energy in each cell (K c )at z ¼0.25h m for various tested discharges:(a)small adults (15<TL <25cm)and (b)large adults (25 TL <35cm)IBERIAN BARBEL’S RESPONSE TO VELOCITY AND TURBULENCE。
The effect of soil water content, soil temperature, soil pH-value and the root mass on

Plant and Soil(2005)268:21–33©Springer2005 DOI10.1007/s11104-005-0175-5The effect of soil water content,soil temperature,soil pH-value and the root mass on soil CO2efflux–A modified modelSascha Reth1,3,Markus Reichstein2&Eva Falge11Department of Plant Ecology,University of Bayreuth,D-95440Bayreuth,Germany.2Potsdam Institute for Climate Impact Research Natural Systems Department,D-14473Potsdam,Germany.3Corresponding author∗Received10February2004.Accepted in revised form21April2004Key words:fine-root mass,non-linear regression model,pH respiration,soil moisture,soil temperatureAbstractTo quantify the effects of soil temperature(T soil),and relative soil water content(RSWC)on soil respiration we measured CO2soil efflux with a closed dynamic chamber in situ in thefield and from soil cores in a controlled climate chamber experiment.Additionally we analysed the effect of soil acidity andfine root mass in thefield.The analysis was performed on three meadow,two bare fallow and one forest sites.The influence of soil temperature on CO2emissions was highly significant with all land-use types,except for onefield campaign with continuous rain. Where soil temperature had a significant influence,the percentage of variance explained by soil temperature varied from site to site from13–46%in thefield and35–66%in the climate chamber.Changes of soil moisture influenced only the CO2efflux on meadow soils infield and climate chamber(14–34%explained variance),whereas on the bare soil and the forest soil there was no visible effect.The spatial variation of soil CO2emission in thefield correlated significantly with the soil pH andfine root mass,explaining up to24%and31%of the variability.A non-linear regression model was developed to describe soil CO2efflux as a function of soil temperature,soil moisture,pH-value and root mass.With the model we could explain60%of the variability in soil CO2emission of all individualfield chamber measurements.Through the model analysis we highlight the temporal influence of rain events.The model overestimated the observedfluxes during and within four hours of the last rain event. Conversely,after more than72h without rain the model underestimated thefluxes.Between four and72h after rainfall,the regression model of soil CO2emission explained up to91%of the variance.IntroductionSoil CO2fluxes are the second major component of the global carbon cycle(Reich and Schlesinger1992), and play an important role in climate change.Very often is it hypothesized that soils provide a positive feedback to climate warming due to the exponential response of soil CO2efflux to temperature(e.g.Cox et al.,2000;Kirschbaum,1995).However,the gas ex-change between the soil and the atmosphere depends on numerous complex and non-linear relationships, like physiological,biochemical,chemical,ecological and meteorological conditions(Jarvis,1995;Schimel et al.,1994).Soil respiration represents the biolog-ical activity of the entire soil biota,including soil ∗FAX No:+490921552061microbes(e.g.bacteria,fungi,algae,protozoa),plant roots and macroorganisms(e.g.earthworms,nema-todes,insects).The rates of soil CO2efflux vary by ecosystem(Reich and Schlesinger,1992)and are the major component of whole-ecosystem respiration,that in turn explains much of the continental gradient of the net carbon balance(Schulze et al.,1999;Valen-tini et al.,2000).So show Kelliher et al.(1999)and Law et al.(1999b)for forested ecosystems,that soil respiration amounts to76–77%of the annual GPP, whereas agricultural crops during fallow periods act as a carbon emitter(Soegaard,1999;Soegaard,et al. 2003).Despite these general trends emissions of CO2 are highly spatially variable within one site(Law et al., 2001;Longdoz et al.,2000;Simek et al.,2004).22A positive correlation between soil temperature and soil CO2efflux is well described by several re-views(Kätterer et al.,1998;Lloyd and Taylor,1994; Reich and Schlesinger,1992;Singh and Gupta,1977). Also,soil moisture affects the soil CO2efflux(Bun-nell et al.,1977;Orchard and Cook,1983;Reichstein et al.,2002;Simek et al.,2004;Subke et al.,2003). Furthermore,soil CO2efflux is influenced by other factors like,substrate amount(Zak et al.,2000),the pH-value of the soil(Hall et al.,1997)as well as the activity of the vegetation(Reichstein et al.,2003b) since root respiration(Janssens et al.,1998;Kutsch et al.,2001;Law et al.,1999a)and heterotrophic res-piration(Goulden et al.,1996;Hollinger et al.,1998) comprise total soil CO2efflux,and plants continu-ously excrete exudates into the soil.Several studies showed significant effects of soil pH values on soil respiration(Andersson and Nilsson,2001;Hall et al., 1997;Sitaula et al.,1995)since,in particular,mi-crobial activity increases with rising pH values(Ellis et al.,1998).Furtheron,temporal effects like litter fall,decom-position dynamics and the amount and the timing of rainfall(Ball et al.,1999;Jackson et al.,1998)influ-ence soil respiration.The effect of rainfall was often larger than expected from the relationship between soil moisture and CO2efflux(Davidson et al.,1998; Russell and V oroney,1998).A series of models try to explain the relation-ship between the factors governing soil CO2ef-flux.Most studies use different principles to describe temperature effects, e.g.linear regression analysis (Witkamp,1966),Q10(Maljanen et al.,2002;Reich and Schlesinger,1992)or power relationship(Kucera and Kirkham,1971),as well as relationships based on the Arrhenius form(Howard and Howard,1979). However,all existing models cannot explain the total variation of the CO2soil efflux.Numerous empirical models were developed for crop(Boegh et al.,1999) and meadow soils(Bremer and Ham,2002)or bare soils(Gupta et al.,1981;Reth et al.,2004).These models are not useful for forest soils.In contrast forest models(Baldocchi and Wilson,2001;Janssens et al., 2001;Nakano et al.,2004;Rasse et al.,2001)are often of low use at bare soil or meadows.The aim of this study is to analyse the influence of soil temperature,moisture,pH value and root mass on soil CO2efflux through a combination offield and lab-oratory experiments.In a second step these effects are assembled into an empirical model that should work on meadow and cropland as well as in forest and bare fallow soil.Finally,we explore a robust regression method to identify temporal effects on soil CO2efflux in thefield that are not represented by the model. MethodsSite descriptionThe measurements used in this study were carried out in the course of special observation periods of the VERTIKO(Vertical transport under complex natural conditions)project,which is part of the AFO2000 (German Atmospheric Research,2000)programme. The target area of the VERTIKO project comprises the region between the Erzgebirge in the South and the Oder-Spree lake district in the North(100km WE and 300km NS).It includes a variety of natural small-scale variability from land use to orographic effects typical for Germany.During three special observation periods (SOPs)measurements were performed at anchor sta-tions located in the target area.For an overview of the parameters observed during thefield campaigns that are expected to influence soil CO2efflux see Table1.The measurements of SOP1were carried out in September and October2001at the Anchor Station Melpitz of the Institute for Tropospheric Research,lo-cated near Melpitz,Saxony(51◦31 N,12◦55 E,86m a.s.l.).The area is aflat managed meadow(MW)of approximately20ha surrounded by farmland(MA, see e.g.Spindler et al.,2001).The annual mean air temperature is8.7◦C and the annual precipitation 539mm.The dominant species were Lolium perenne, Taraxacum officinale and Leontodon autumnalis.The leaf area index(LAI)was2.0m2m−2.The SOP2experiment took place in June and July2002at the Falkenberg Boundary-Layer mea-surement site of the German Meteorological Service, the Lindenberg observatory,Brandenburg(52◦10 N, 14◦07 E,73m a.s.l.).The landscape in this region was formed by inland glaciers of the last ice age, with a slightly undulating orography and a hetero-geneous land use structure(see e.g.Beyrich et al., 2002).The Falkenberg site itself isflat and consists of about18ha of managed meadow(LW)with short grass.An area of approximately3ha of the meadow was ploughed during the experiment(LA).The annual mean air temperature is8.6◦C and the annual precip-itation560mm.Main species were Lolium perenne, Bromus hordeaceus,Festuca rubra,Leontodon autum-nalis,Taraxacum officinale,Trifolium pratense and23 Table1.Observed range of the parameters T soil(soil temperature),RSWC(relative soil watercontent),pH and RRM(fine root biomass,d.w.based)expected to influence soil CO2emissionduring thefield campaigns(MW=Melpitz Meadow,MA=Melpitz Agricultural Fallow,LW=Lindenberg Meadow,LA=Lindenberg Agricultural Fallow,TW=Tharandt Meadow,TF=Tharandt Forest)T soil T soil RSWC RSWC pH pH RRm RRMmin(◦C)max(◦C)min(%)max(%)min max min(%)max(%)MW10.316.6398 5.87.10.0359MA10.721.53999 6.97.400LW14.325.81738 4.5 6.90.4126LA14.518.91617 5.0 5.900TW11.520.95897 5.0 5.526TF9.018.65681 3.3 3.80.3636Trifolium repens.Meadow LAI showed a spatial gra-dient during thefield campaign,with maximum LAI of4.7,and minimum LAI of1.3m2m−2.The SOP3measurements were performed in May and June2003at the Anchor Station Tharandter Wald of the Technical University Dresden near Tharandt, Saxony(50◦58 N,13◦34 E,375m a.s.l.).The slightly undulating experimental area is located inside a closed forest of approximately6000ha.The annual mean air temperature is7.6◦C and the annual precipita-tion820mm.The forest(TF)is dominated by114 years old,approximately28m high Picea abies(L.) KARST trees.The projected leaf area index(LAI) was6.9m2m−2.The meadow area(TW)of the anchor station is1.5ha and dominated by Rumex ob-tusifolium(L.),Holcus lanatus(L.),Cirsium arvense (L.)and Carex spp.The leaf area of the meadow was 2.6m2m−2at the beginning(May,22)of theflux measurements and increased to6.1m2m−2at the end of the campaign(June,13).Thesefield measurements were complemented by climate chamber experiments to extend the range of soil temperatures and soil moisture observed during the SOPs.Soil efflux and soil analysis in thefieldSoil CO2efflux was measured with a non-steady-state flow-through chamber system,andfluxes were deter-mined from the concentration increase in closed cham-bers.The system consists of cylindrical steel chambers (height80mm and diameter197mm)with plexi glass lids attached during the measurement.No fan was used in the system.Overheating could be avoided because single measurements were completed within12min,and soil temperatures inside and outside the chamber differed less than0.2◦C.The chambers were inserted 2cm into the soil and all plant material was removed from the chambers’interiors.Thefirstflux measure-ments started approximately12h after plant cutting to avoid effects on soil CO2efflux by collar inser-tion or plant cutting.Collars remained in place during all subsequent measurements at the site.Ten cham-bers were installed as spatial replicates at each land use type,except at the bare soil of Lindenberg with onlyfive chambers.Measurements took place from the early morning to late in the evening.The chambers were moved to another site or land use afterfinishing 5to14measurements at the same point.The sys-tem allowed to measurefive chambers alternately with magnetic valves controlling theflow of the different chambers.For the concentration measurements the air was pumped in a closed loop from the chamber to the analyser(Photoacoustic Multi-gas Monitor,INNOV A 1312)and back to the chamber.Through a20m long tube with an inside diameter of3mm,the air was sucked for approximately30s with a speed of4m s−1. The concentrations of CO2(for control)and water were determined from the air stream.The CO2efflux was determined from the slope of the concentration increase within a chamber using four concentrations measured at238s intervals.The system was tested against other measurement systems(non-steady-state flow-through chambers,non-steady-state non-flow-through chambers,non-steady-state non-flow-through chambers and a calibration system)in a calibration experiment(Pumpanen et al.,2004).In this experi-ment he system employed showed an underestimation of approximately4%for soils comparable to those in this study,and at maximum11%for dryfine sand.24Parallel to the soilflux measurements environ-mental parameters were observed quasi-continuously. Soil temperature(Thermistor,Siemens M841)was recorded at2cm depth inside each chamber and out-side the chambers every5min.V olumetric soil water content(SWC,m3water per m3total soil volume, Theta Probe,ML2)was measured half hourly in the upper10cm of the soil at each stand.Relative soil wa-ter content(RSWC)is defined as SWC divided byfield capacity,allowing for a better comparison of soils with different textures.Reichstein et al.(2002)found very similar RSWC1/2parameters for a sandy and clayey soil,Nevertheless one would not expect exactly the same values of RSWC1/2in all soils,and our assump-tion that reduces the number of model parameters introduces(albeit little)model error.Analysis of root mass and pH of soil samples of each chamber were performed afterfinishingflux measurements.Soil cores with a diameter of5cm and a depth of10cm were taken in thefield,and soil and roots were separated manually.The remaining soil was sieved to remove stones.The root biomass was dried three days at105◦C.The dry mass of the roots was expressed per unit dry mass of the oven-dry soil.The pH-value was determined from a fresh soil slurry using a glass electrode(Scheffer and Schachtschabel,2002). Incubation time of20g soil was1h in50g distilled water.Soil efflux in the climate chamberFor the climate chamber experimentsfive replicate fresh soil cores(diameter=31cm,height=25cm) were collected under minimal disturbance from the meadow of Melpitz,Lindenberg and Tharandt as well as from the fallow of Lindenberg and the forest of Tharandt.CO2efflux of the soil samples was recorded over112days.Soil temperature was manipulated by changing the air temperature inside the climate chamber.Starting at20◦C the soil temperature was decreased every two days by2◦C.After reaching4◦C the soil temperature was increased every two days by 2◦C up to38◦C,then decreased again and so forth. Soil water content was altered by irrigation and drying cycles.At beginning and end of eachflux measure-ment(for description of the system see above),we weighed the soil cores for gravimetric determination of soil water content.In the climate chamber it was not possible to analyse the soil without destroying the soil cores.Therefore the soils were not analysed for the parameters pH and RRM.Soil CO2modelThe non-linear regression model of soil CO2efflux was adapted from Reichstein et al.(2003b,2002)that includes a function for soil temperature(T soil,Equa-tion3)–following an exponential response,a function of relative soil water content(RSWC,Equation4) and a function of vegetation activity.We modified the model by Reichstein(2003b)for better incorporation of the actually measured data in two ways:(1)The soil CO2emission rate under standard conditions,was made dependent on root mass per soil mass as a proxy for vegetation activity(instead of leaf area index in Reichstein,et al.2003b);(2)We included the influ-ence of soil chemistry through including the soil pH as additional predictor.Mathematically,the model is described by the following equations:R soil=R ref∗F(T soil)∗g(RSW C)∗h(pH),(1) where R soil is the soil CO2efflux.The emission under standard conditions(R ref),at T ref and non-limiting soil water content,is described by:R ref=H resp+a resp,(2) where h resp represents heterotrophic respiration and a resp autotrophic respiration.The heterotrophic respi-ration is afitted parameter and a resp is a linear function of the root mass per dry soil mass(RRM)and a parameter(rf):a resp=R RM∗r f.(3) The exponential increase of the CO2emission with soil temperature is described by:f(T soil)=expE0∗1T ref−T0−1T soil−T0,(4) where E0is a free parameter analogue to the activation energy in the standard Arrhenius model,T ref is the reference soil temperature and T0the lower temper-ature limit for R soil.T ref was set to15◦C and T0at −46.02◦C(Lloyd and Taylor,1994).The response of changes on relative soil water content is described by: g(RSW C)=RSW C−RSW C0(RSW C1/2−RSW C0)+(RSW C−RSW C0).(5)RSWC1/2represents the RSWC at half-maximum soil CO2efflux and RSWC0is the residual soil water con-tent,below which the efflux ceases.RSWC1/2and RSWC0are free parameters.Finally,the response of the CO2emission with soil pH-value follows an optimum curve:25 Table2.Coefficients of variation for the univariate analysis of soil CO2emission influencing parametersduring thefield(FM)and the climate chamber measurements(CCM).T soil(soil temperature),RSWC(relative soil water content),pH and RRM(fine root biomass,d.w.based),n represents the number ofCO2flux observations not affected by rain,and used for the regression model parameterisation.For siteabbreviations see Table1FM CCMT soil RSWC RRM pH n T soil RSWCMW0+0+0+0+00.45∗0.1MA0+0+ND0+00.35∗0.09LW0.25∗0.34∗0.12∗0.19∗1410.52∗0LA0.46∗0ND0.24∗30ND NDTW0.31∗0.18∗0.3∗0.19∗500.65∗0.14∗TF0.13∗0.040.31∗0.23∗740.66∗0P<0.01,ND=not determined.+not used for model parameterisation.h(pH)=exp−pH−phOptphSens2,(6)where phOpt is a free parameter and represents the optimal pH value.The parameter phSens describes the sensitivity of soil CO2efflux to deviation from this optimal value.Parameter estimationFor the data analysis with the non-linear regression model we used a robust regression technique that is able to objectively identify outliers,or more pre-cisely data points,that are inconsistent with the model assumptions.We used the non-linear least trimmed squares(LTS)regression(Reichstein et al.,2003a; Stromberg,1997),that seeks to minimize the sum of squared residuals as ordinary non-linear regression, but with exclusion of the largest x%of residuals,that are assumed to be due to contaminated data or due to data inconsistent with the model.Formally the objec-tive function that has to be minimised is the trimmed sum of squared errors(TSSE):T SSE=i≤N·(1−0.001−t)r2i,(7)where r i is the i-th smallest residual,N is the to-tal number of data points,and(0.01t)is the fraction of residuals to be excluded.The procedure was per-formed with trimming percentages of10,20,30% and subsequently analysed which data was classified as‘contaminated’by the procedure.ResultsWe examined the effect of soil temperature changes on CO2efflux at the four soil types of thefield mea-surements and offive soil types in the climate chamber experiment.Due to the continuous rain during SOP1, the results of thefield measurements in Melpitz were not used in the temperature,and all further analyses. An exponential increase with increasing soil tempera-ture was observed at all soils(Figure1),both during thefield measurements and in the climate chamber experiment(Table2).Meadow soils,except MW in bothfield and cli-mate chamber measurements,and LW in the climate chamber measurements,responded to changes of rel-ative soil water content.There was no statistically significant effect at the fallow and the forest soil,both in thefield and in the climate chamber measurements (Table2).Variation of the pH-value of the soils and between the single measurement points at each site showed a positive correlation with the CO2efflux(Figure2). During simultaneous measurements with similar soil temperature and soil water content,the chambers with higher soil pH exhibited higher CO2fluxes,except in Melpitz(Table2).Also the presence offine roots significantly af-fected the observed soil CO2efflux.At all meadow and forest stands,again except for Melpitz,the relative root mass was correlated positively(Figure3)with the CO2flux rates(Table2).While the univariate relationships between soil CO2efflux and environmental factors were gener-ally weak,the above soil CO2efflux model already26Figure1.Soil CO2efflux as an exponential function of soil temperature(T soil)for the meadow soil of Tharandt(TW)in thefield(closed dots, r2=0.31),and in the climate chamber(open dots,r2=0.65).The lines are regression lines,calculated from the used data using equation4.Figure2.Soil CO2efflux as function of soil pH-value at the fallow in Lindenberg(LA).Dots represent the mean CO2fluxes(n=5)with error bars(r2=0.24).The line is the regression line,calculated from the used data using equation6.27 Figure3.Soil CO2efflux as function of relative root mass(RMM)at the meadow soil of Tharandt(TW).Dots represent the mean CO2fluxes (n=6)with standard deviation(r2=0.30).The line is the regression line,calculated from the used data using equation3.explained60%of the variability of soil CO2efflux (Figure4a).With the robust regression approach we analysed inconsistence of the model(Figure4b–d). 30%of the data could be identified as inconsistent with the model and could be related to disturbance by precipitation events(Figure4e).Thereby we could identify3periods:During and up to4h after a rain event(period1)the model overestimated the measured CO2fluxes.In contrast, after a dry period of more than72h(period2)the model underestimated thefluxes.In the time period in between,that is4to72h after the last rain event (period3),the model reflected the measured emissions well,and explained91%of their variability(Table3). Interestingly,the amount of rain did not affect the per-formance of the model.The robust regression method rejected87%of the data points falling into period1or 3,supporting the rationale to identify and exclude data that are inconsistent with the model.DiscussionIn this paper we confirmed well known correlations of soil CO2efflux and abiotic factors,although some-times the ranges of driving forces in thefield were too small to detect previously reported effects,e.g.on Q10.The strong correlation of temperature and soil CO2 emission was quantified for many soils under different conditions(see e.g.Epron et al.,1999;Kätterer et al., 1998;Lloyd and Taylor,1994;Reich and Schlesinger, 1992).In our climate chamber measurements,all soils showed an exponential increase of soil CO2efflux with increasing soil temperature(P<0.01).In the field the soil CO2efflux was more variable,indicating increasing influence of other parameters.A similar distinction was observed comparing soil CO2exchanges at changing soil moisture.Only at the meadow stands in Tharandt and in thefield measure-ments of Lindenberg soil CO2efflux showed signifi-cant(P<0.01)response to soil moisture changes.At the other stands,and partly during the climate chamber experiments,the relative soil water content span al-lowed only for small limiting effects due to soil water. Also,Reichstein et al.(2003b)observed a broad range28parison of modeled and measured soil CO2efflux:(a)without a trimmed fraction(r2=0.60),(b)with a trimmed fraction of 10%(r2=0.80),(c)with a trimmed fraction of20%(r2=0.86),(d)with a trimmed fraction of30%(r2=0.91)and(e)evaluating the temporal effect of last rain event.Lines are1:1lines.29 Table3.Parameters of the nonlinear regression model for all stands with a trimmed fraction of30%(n=295).240values were not effected by rain or drought,and indicated as consistentwith the model(LW:n=97,LA:n=30,TW:n=44,TF:n=69).Root mean squareerror(RMSE)of the model results was0.89µmol m−2s−1.To take into account the4%underestimation of the system(Pumpanen et al.,2004),the parameters h resp and rf have to bemultiplied with the correction factor0.96Parameter Units Value Standard errorh respµmol m−2s−19.11 2.12E0K247.7816.84RSWC0%92RSWC1/2%170.9phOpt9.35 1.23phSens−4.870.69rfµmol m−2s−1g d.w.19.91 5.23Soil(g d.w.root)−1of near optimum soil water content where changes in soil moisture have little or no effect and correspond to our observations.At the bare soil of SOP2at Linden-berg the soil water content was nearly constant while the measurements were performed,so there was no effect of soil moisture changes on CO2efflux at this time.Even when taking soil temperature and water con-tent into account,the spatial variation of soil CO2 efflux at one site can be large.Buchmann(2000)re-ported spatial variations among soil collars,which were larger than the diurnal variability of soil CO2 emission rates measured with the same collars during a day.This corresponds well with ourfield measure-ments,in particular at the forest stand,where soil temperature changes were very small,but spatial vari-ability was high.Thus,multivariate interaction of various other factors has to be accounted for as in the model presented here.The link of respiration to vegetation productivity established by Reichstein et al.(2003b)with poten-tially confounding factors at the continental scale,was here confirmed at small scale for soil CO2efflux.An-derson(1992)and Janssens et al.(1998)showed,that root respiration may account for half of the soil efflux. In general,this agreed with our observations for the forest and meadow sites.In addition,samples with higher root mass per soil showed higher CO2emission (P<0.01)at comparable meteorological conditions. Thisfinding held within a site,but not among differ-ent sites,where other factors determined the overall magnitude of soil CO2efflux.An influence of spatial heterogeneity of soil pH on soil CO2emission was confirmed at all stands (P<0.01),except Melpitz.Several studies described a similar positive correlation of pH-value and soil CO2 efflux(Andersson and Nilsson,2001;Ellis et al.,1998; Hall et al.,1997;Sitaula et al.,1995).Baath(1996) and Högberg et al.(2003)demonstrated the direct pos-itive effect on soil respiration with pH tolerance of the bacterial community.A biological activity of soil microorganisms is permitted between a soil pH of a minimum of3and a maximum of7to8(Scheffer and Schachtschabel,2002).Between these values(see Ta-ble1),and otherwise constant conditions we observed a nearly linear increase of soil CO2emission.In the model however,we described the response to soil pH with an optimum curve to account for potential decline in soil CO2emission above a pH of9.Similar pattern were shown in Wittmann et al.(2004)with an opti-mum curve for the dependence of hydrolytic enzyme activities in a forest soil.The nonlinear regression model gave good results for all investigated sites.Up to60%of the data vari-ance could be explained by soil temperature,relative soil water content,soil pH and relative root mass. Evaluating the time span between measurement and last occurring rain,the modeled soil CO2effluxes overestimated the measuredfluxes in the case of rain or maximum4h after the last rain.The main cause for this could be the reduction of the soil air-filled pore space resulting in reduced gaseous diffusivities. The negative effect of waterfilled pores on soil CO2 emission is often discussed in the literature(see e.g. Ball et al.,1999;Lee et al.,2002).30After three or more days without rain,the model underestimated the observedfluxes.An explanation for this might be a shift of the main respiratory ac-tivity to deeper soil layers,with soil moisture and temperatures more favourable to respiration than those recorded by the sensors in the top soil layer.Another explanation could be thatfine roots dying in the upper soil,and new root development in deeper soil layers led to an increase in CO2release.For the time be-tween4and72h after a rain event,the model worked well,explaining91%of the soil efflux variation with changes in soil temperature,soil water content,root mass and soil pH.Potential limitation of the model could be that the temperature,soil water content,and pH responses of respiration arising from roots and soil heterotrophs might differ.Root respiration depends on current pho-tosynthetic products as substrate,and is therefore mainly controlled by light availability during the last2 days(Fitter et al.,1998).Heteorotrophs use older pho-tosynthetic products(e.g.litter,turnover offine roots), but also use root exudates(Grayston et al.,1997),as rhizosphere micro-organisms rapidly acquire the iso-topic signature of the current photosynthate(Pendall et al.,2003),therefore being partly coupled to light availability too.In our case,we had to simplify these effects,as it was not possible to separate the responses of these two componentfluxes from data measured with our technique.We included only the relative amounts of autotrophic and heterotrophic respiration in the model,and applied identical temperature,soil water content,and pH functions.We tried to overcome the limiting effects of rel-atively short measurement campaigns at the various sites with soil cores taken to the climate chamber for wider ranges of temperature and moisture.However, this setup still did not allow for proper assessment of threshold events or sudden shifts in key variables de-termining soil respiration or soil CO2efflux.Yet in the field,Jensen et al.(1996),Lee et al.(2002)and Rey et al.(2002)observed a steep increase of CO2efflux with thefirst rain after drought,indicating dynamic effects on soil CO2efflux.However,with our method we could identify periods in ourfield data set that were not consistent with our static model by the robust re-gression approach.As we removed aboveground plant material before the measurements of soil CO2efflux, and determined only root biomass,we were not able to include the dynamic effects of root activity,or current photosynthates on root and heterotrophic respiration.Due to these limitations our model might be re-stricted from its formulation and parameterisation to finer time scales,yet we believe that the model can be used for long-term predictions(up to a year), when coupled to a prognostic model for soil moisture, temperature,fine root biomass and pH.The model equations per se do not allow for feedbacks,dynamic responses or nonlinear(sensu strictu)events,but could enhance current generation carbon cycle mod-els,which mainly concentrate on temperature effects (e.g.Cox et al.,2000),with additional factors as soil moisture,fine root biomass and pH,to help address complex ecological relationships to identify feedbacks between soil respiration and climate change.We have shown that the robust regression approach is very useful as an objective means of ecological data analysis,when carefully interpreted.Through this approach we obtained parameters that are valid for normal conditions and that describe the data very well, while at the same time highlighting model problems under non-normal,transient conditions,namely dur-ing or shortly after rain events or after longer periods (>72h)of dry conditions.With a standard regression approach on the contrary,one would have got average, effective parameters that are affected by the conditions under which the model is not valid,and thus are‘fitted’parameters in the bad sense of the word.The robust regression approach helps to avoid including periods in the parameterisation that are beyond the scope of the model,e.g.transient changes in diffusion path-ways or location and status of biological activity and lead to unwanted errors even in the range where the model could be valid.Moreover,we determined4to 72h as the time scale for our investigated systems, where a model based on steady-state conditions is suit-able when accounting for changes in soil temperature, moisture,pH andfine root biomass.ConclusionIn this study we developed a model that allows esti-mation of soil CO2efflux on bare soils,meadow soils as well as forest soils.The study confirmed soil tem-perature and soil water content as the most important factors influencing soil CO2emission.In addition soil pH and relative root mass are found as important fac-tors to describe spatial variation of soil CO2emission due to vegetation productivity and microbial activity spans.。
THE EFFECT OF WATER CONTENT ON THE MECHANICAL BEHAVIOUR OF FINE-GRAINED SEDIMENTARY ROCKS
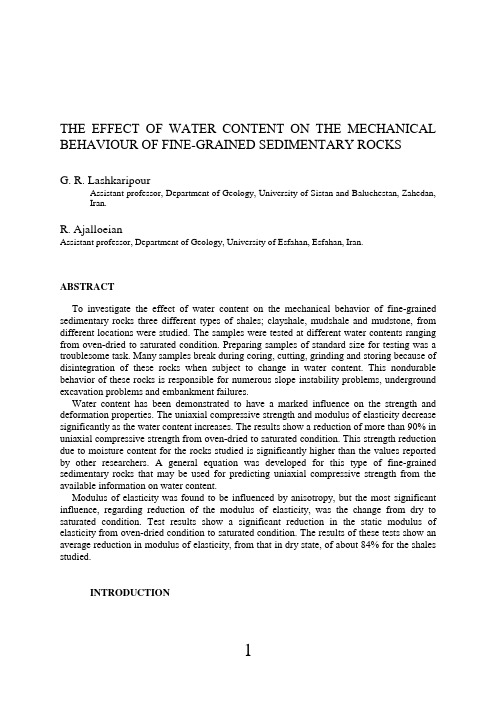
THE EFFECT OF WATER CONTENT ON THE MECHANICAL BEHAVIOUR OF FINE-GRAINED SEDIMENTARY ROCKSG. R. LashkaripourAssistant professor, Department of Geology, University of Sistan and Baluchestan, Zahedan, Iran.R. AjalloeianAssistant professor, Department of Geology, University of Esfahan, Esfahan, Iran.ABSTRACTTo investigate the effect of water content on the mechanical behavior of fine-grained sedimentary rocks three different types of shales; clayshale, mudshale and mudstone, from different locations were studied. The samples were tested at different water contents ranging from oven-dried to saturated condition. Preparing samples of standard size for testing was a troublesome task. Many samples break during coring, cutting, grinding and storing because of disintegration of these rocks when subject to change in water content. This nondurable behavior of these rocks is responsible for numerous slope instability problems, underground excavation problems and embankment failures.Water content has been demonstrated to have a marked influence on the strength and deformation properties. The uniaxial compressive strength and modulus of elasticity decrease significantly as the water content increases. The results show a reduction of more than 90% in uniaxial compressive strength from oven-dried to saturated condition. This strength reduction due to moisture content for the rocks studied is significantly higher than the values reported by other researchers. A general equation was developed for this type of fine-grained sedimentary rocks that may be used for predicting uniaxial compressive strength from the available information on water content.Modulus of elasticity was found to be influenced by anisotropy, but the most significant influence, regarding reduction of the modulus of elasticity, was the change from dry to saturated condition. Test results show a significant reduction in the static modulus of elasticity from oven-dried condition to saturated condition. The results of these tests show an average reduction in modulus of elasticity, from that in dry state, of about 84% for the shales studied.INTRODUCTIONWater content in fine-grained sedimentary rocks is an important consideration in many engineering projects. It has been well established that water content of these rocks significantly changes most aspects of physical and mechanical properties. Fine-grained sedimentary rock in this study includes mudrock or shale. This type of rock is formed in both marine and nonmarine environments and it is one of the most abundant sedimentary rocks. Tucker (1991) reported that mudrock is the most abundant of all lithologies, constituting some 45-55% of sedimentary rock sequences. Because of its abundance, mudrock is the most frequently encountered rock type in engineering construction. King et al. (1994) reported that shales are found in many large civil engineering structures in United Kingdom and elsewhere. Moreover this rock is one of the most problematic materials for geotechnical and petroleum engineers.Many investigators have studied the behavior of fine-grained sedimentary rock under different water content conditions. (Van Eeckhout, 1976; Bauer, 1984; Tandanand, 1985; Steiger & Leung, 1990; Zhiyl & Jinfeng, 1993; Hsu & Nelson, 1993; Ghafoori, 1995; Lashkaripour & Ghafoori, 1999). Analysis of their results has shown that water content of these rocks significantly changes most aspects of physical and mechanical properties.Van Eeckhout (1976) studied the effect of water content on the strength of different rocks. He found a significant reduction in the strength of shale due to an increase in water content from dry condition to saturated condition. He lists five processes of strength loss in shales due to increased moisture content: fracture surface energy reduction, capillary tension decrease (equivalent to the air-breakage of Terzaghi & Peck, 1967), pore pressure increase, frictional reduction, and chemical deterioration.Hsu and Nelson (1993) reported a strong correlation between compressive strength and water content for Cretaceous clay shales of north America. Steiger and Leung (1990) reported that, in shales, unconfined compressive strengths measured with dry samples can be 2 to 10 times higher than for wet samples. Colback and Wiid (1965) found that the compressive strength of quartzitic shale under saturated condition was about 50% of that under dry condition.EXPERIMENTAL RESULTS AND DISCUSSIONTo investigate the effect of moisture content on the mechanical behavior of fine-grained sedimentary rock, mudrocks from different locations were tested. The main aim of these series of tests was to establish a correlation between the results of uniaxial compression tests and values of water content.Preparing samples of standard size (NX=54mm) for testing was a troublesome task. These samples were obtained from the blocks of mudrocks from fresh excavation. Many samples break during coring, cutting, grinding and storing because of disintegration of this rock when subject to change in water content and an inherent weakness of bedding planes. This causes great difficulties in sample preparation.All specimen for this research were prepared in the direction perpendicular to the laminations.Effect of Water Content on the StrengthTo study the effect of water content on the strength of mudrock, three types of mudrocks were tested at different water contents varying from oven-dried to saturated condition. Table 1 shows the results of compression tests of mudrocks at different water content. This tablepresents a reduction of more than 90% in compressive strength from oven-dried to saturated condition. This strength reduction due to moisture content for the mudrocks studied is significantly higher than the values reported by other researchers.The results of these tests have been presented graphically in figures 1(a), 1(b) and 1(c). These figures show a strong correlation to an exponential relationship between uniaxial compressive strength and water content.Equations for the plots in Figure 1 are as follows:σc= 88.087 e-0.544w(1a) σc= 88.781 e-0.44w(1b)σc= 82.279 e-0.411w(1c) where σc= uniaxial compressive strength in MPaw = water content in %.The same trend of decreasing compressive strength with increasing water content was found for all three types of mudrock tested as shown by overlaying the results in Figure 2. A correlation was made for all tested mudrocks in Figure 3 and a general equation was drived for mudrocks (including clayshale, mudshale and mudstone) as follows:σc= 83.592 e-0.443w(2)As shown in equation 2, the water content significantly affects the uniaxial compressive strength of the mudrocks. From equation 2 a general equation was developed to use for the mudrocks as follows:Figure 1. Effect of water content on the uniaxial compressive strength of tested mudrocks; (a)Figure 2. Trend of decreasing compressive strength with increasing water content for all testedmudrocks.Figure 3. Correlation between uniaxial compressive strength and water content for tested mudrocks.σc= σdry e-0.443w(3) where σc= uniaxial compressive strength at variable water content in MPa,σdry= uniaxial compressive strength at dry condition in MPa.It seems that different parameters control strength loss in mudrocks due to increasing moisture content as noted by Van Eeckhout (1976). Also, mudrock is more affected by the addition of water, especially in the case of montmorillonite clayshale.Effect of Water Content on the Deformation PropertiesModulus of elasticity was found to be influenced by anisotropy but the most significant influence, regarding reduction of the modulus of elasticity, was the change from dry condition to saturated condition. A comparison between calculated values of static modulus of elasticity for oven-dried, air-dried, and saturated conditions for three types of fine-grained sedimentary (clayshale, mudshale and mudstone) is presented in Figure 4. This figure shows a significant reduction in the static modulus of elasticity from oven-dried condition to saturated condition. The results show an average reduction in modulus of elasticity in relation to that of the dry state of about 84% for these samples. The highest value of static modulus of elasticity reduction was associated with the mudshale samples.Figure 4. Comparison of static modulus of elasticity for oven-dried, air-dried and saturatedconditions for tested mudrocks.The test results show that water content can significantly affect the mechanical behavior (such as strength and deformation properties) of mudrocks.CONCLUSIONSSample preparation for testing was a difficult task in this study. A large number of the uniaxial compressive strength test samples disintegrated upon contact with water or cracked during drying in oven.Water content has been demonstrated to have a marked influence on the mechanical behavior, such as strength and deformation properties, of these rocks. Testing has shown significant decrease in the uniaxial compressive strength and modulus of elasticity as the water content increases. These strength and modulus of elasticity reductions for the mudrocks studied are significantly higher than the values reported by other researchers.A general equation was developed for the mudrock that may be used for predicting uniaxial compressive strength from the available information on water content and dry uniaxial compressive strength for this type of rocks.REFERENCESBauer, R.A. 1984.The relationship of uniaxial compressive strength to point load and moisture content indices of highly anisotropic sediments of the Illinois basin. Proc. 25th US Symp. on Rock Mech., Northwestern University: 398-405.Colback, P.S.B. & Wiid, B.L. 1965.The influence of moisture content on the compressive strength of rocks. Proc. 3rd Can. Rock. Mech. Symp., Toronto: 55-83.Ghafoori, M. 1995.Engineering behaviour of Ashfield shale. PhD thesis, University of Sydney. Australia.Hsu, S.C. & Nelson, P.P. 1993.Characterisation of Cretaceous clay shales in North America. Proc. Int. Symp. on Geotech. Eng. of Hard Soils-Soft Rocks, Anagnostopoulos et al. (eds), Balkema, Rotterdam: 139-146.King, M.S., Andrea, M.0. & Shams Khanshir, M. 1994.Velocity anisotropy of Carboniferous mudstones. Int. J. Rock Mech. Min. Sci. & Geomrch. Abstr. 31: 261-263. Lashkaripour, G.R. & Ghafoori, M. 1999.Predicting mechanical properties of shale from index parameters. Proc. 2nd Asian Symp. on Engineering Geology and the Environment. Bangi, Malaysia: 2-35 to 2-39.Steiger, R.P. & Leung, P.K. 1990. Predictions of wellbore stability in shale formations at great depth. In: Maury, V. & Fourmaintraux, D. (eds), Rock at great depth, Balkema, Rotterdam: 1209-1218.Tandanand, S. 1985.Moisture adsorption rate and strength degradation of Illinois shales. Proc. 26th US Symp. on Rock Mech., Rapid City: 595-600.Terzaghi, K. & Peck, R.B. 1967.Soil mechanics in engineering practice, 2nd ed. John Wiley and Sons, New York, 729 p.Tucker, M.E. 1991. Sedimentary petrology, an introduction to the origin of sedimentary rocks, 2nd ed. Blackwell Scientific Publications, London, 260 p.Van Eeckhout, E.M. 1976. The mechanisms of strength reduction due to moisture in coal mine shales. Int. J. Rock Mech. Min. Sci. & Geomech. Abstr. 13: 61-67.Zhiyl, L. & Jinfeng, W. 1993. Engineering properties of Chongqing Mudstone, China. Proc. 26th Annual Conf. of the Eng. Group of the Geol. Soci., The Engineering Geology of Weak Rocks, Cripps et al. (eds), Leeds: 101-107.。
EFFECTS-OF-AEROSOLS-ON-AUTUMN-PRECIPITATION-OVER-MID-EASTERN-CHINA
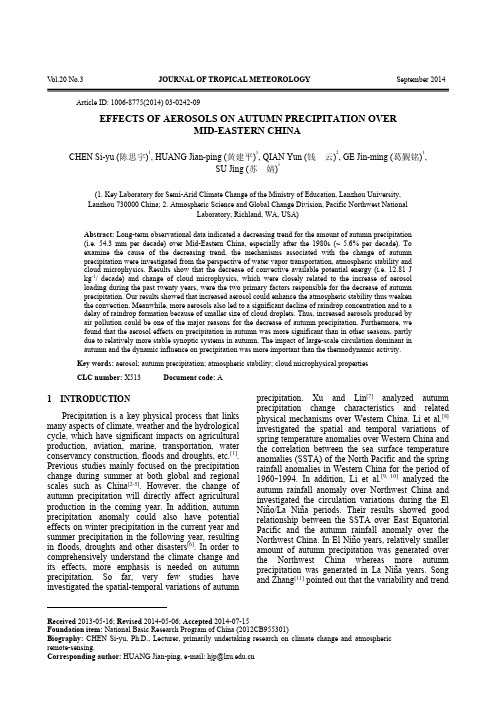
V ol.20 No.3 JOURNAL OF TROPICAL METEOROLOGY September 2014Article ID: 1006-8775(2014) 03-0242-09EFFECTS OF AEROSOLS ON AUTUMN PRECIPITATION OVERMID-EASTERN CHINACHEN Si-yu ( )1, HUANG Jian-ping ( )1, QIAN Yun ( )2, GE Jin-ming ( )1,SU Jing ( )1(1. Key Laboratory for Semi-Arid Climate Change of the Ministry of Education, Lanzhou University, Lanzhou 730000 China; 2. Atmospheric Science and Global Change Division, Pacific Northwest NationalLaboratory, Richland, WA, USA) Abstract: Long-term observational data indicated a decreasing trend for the amount of autumn precipitation (i.e. 54.3 mm per decade) over Mid-Eastern China, especially after the 1980s (~ 5.6% per decade). To examine the cause of the decreasing trend, the mechanisms associated with the change of autumn precipitation were investigated from the perspective of water vapor transportation, atmospheric stability and cloud microphysics. Results show that the decrease of convective available potential energy (i.e. 12.81 J kg -1/ decade) and change of cloud microphysics, which were closely related to the increase of aerosol loading during the past twenty years, were the two primary factors responsible for the decrease of autumn precipitation. Our results showed that increased aerosol could enhance the atmospheric stability thus weaken the convection. Meanwhile, more aerosols also led to a significant decline of raindrop concentration and to a delay of raindrop formation because of smaller size of cloud droplets. Thus, increased aerosols produced by air pollution could be one of the major reasons for the decrease of autumn precipitation. Furthermore, we found that the aerosol effects on precipitation in autumn was more significant than in other seasons, partly due to relatively more stable synoptic systems in autumn. The impact of large-scale circulation dominant in autumn and the dynamic influence on precipitation was more important than the thermodynamic activity. Key words: aerosol; autumn precipitation; atmospheric stability; cloud microphysical properties CLC number: X513 Document code: AReceived 2013-05-16; Revised 2014-05-06; Accepted 2014-07-15Foundation item: National Basic Research Program of China (2012CB955301)Biography: CHEN Si-yu, Ph.D., Lecturer, primarily undertaking research on climate change and atmospheric remote-sensing.Corresponding author: HUANG Jian-ping, e-mail: hjp@1 INTRODUCTIONPrecipitation is a key physical process that links many aspects of climate, weather and the hydrological cycle, which have significant impacts on agricultural production, aviation, marine, transportation, water conservancy construction, floods and droughts, etc.[1]. Previous studies mainly focused on the precipitation change during summer at both global and regional scales such as China [2-5]. However, the change of autumn precipitation will directly affect agricultural production in the coming year. In addition, autumn precipitation anomaly could also have potential effects on winter precipitation in the current year and summer precipitation in the following year, resulting in floods, droughts and other disasters [6]. In order to comprehensively understand the climate change and its effects, more emphasis is needed on autumn precipitation. So far, very few studies have investigated the spatial-temporal variations of autumn precipitation. Xu and Lin [7] analyzed autumn precipitation change characteristics and related physical mechanisms over Western China. Li et al.[8] investigated the spatial and temporal variations of spring temperature anomalies over Western China and the correlation between the sea surface temperature anomalies (SSTA) of the North Pacific and the spring rainfall anomalies in Western China for the period of 1960 1994. In addition, Li et al.[9, 10] analyzed the autumn rainfall anomaly over Northwest China and investigated the circulation variations during the El Niño/La Niña periods. Their results showed good relationship between the SSTA over East Equatorial Pacific and the autumn rainfall anomaly over the Northwest China. In El Niño years, relatively smaller amount of autumn precipitation was generated over the Northwest China whereas more autumn precipitation was generated in La Niña years. Song and Zhang [11] pointed out that the variability and trendNo.3 CHEN Si-yu ( ), HUANG Jian-ping ( ) et al. 243243of autumn precipitation are more significant than that in other seasons during the 20th century over the Northwest China. Zhang et al.[12] found that the change of general atmospheric circulation and the influence of ENSO events under global warming could be the direct factors in effecting the autumn precipitation generation over Northwest China. Shi [13] found a good relationship between precipitation anomaly in autumn and rainfall in the flood season of the following year. However, the above studies were limited only to the Northwest China region, with a focus on the interactions between autumn precipitation and natural climate variability (e.g., ENSO) at large scale. In this study, we investigated the causes of the autumn precipitation anomalies from the perspective of physical mechanisms influencing the precipitation change over Mid-Eastern China.Generally speaking, there are three dominant conditions for the formation of precipitation: (1) Horizontal transportation: water vapor is transported to the precipitation zones from source regions; (2) Convergence and upward movement: the strong upward motion is associated with the surface convergence over the precipitation regions. Water vapor condenses into cloud droplets or ice crystals in the adiabatic expansion during the lifting process. (3) Microphysical conditions with growth of the cloud droplets: The saturated air tends to condense on condensation nuclei and then water droplets grow, become heavier, and fall to the ground as precipitation [1]. Aerosol particles, which have close relationship with the last two points of conditions, are the dominant factors for the formation of precipitation [14-16]. Based on the observational data and modeling approach, Qian et al.[17] found a decreasing trend of light rain events over North America, Europe and Asia from 1973–2009, especially over East Asia, where a remarkable shift from light to heavy rain events has been observed. Besides, Qian et al.[18] pointed out that aerosols are at least partly responsible for the decreasing frequency and amount of light rain in summer over Eastern China. Huang et al.[19, 20] found that dust could heat cloud droplets, increase the evaporation of cloud droplets and further reduce the cloud water path, which may play an important role in cloud development and contribute to the reduction of precipitation over arid and semi-arid areas. Zhao et al.[21] also found a positive feedback mechanism between increased aerosol loadings and reduced precipitation over Mid-Eastern China. Based on 194 observation stations, Gong et al.[22] investigated the frequency change of daily precipitation in summer during 1979–2002 and found an obvious weekly cycle effects.Was the change of autumn precipitation over Mid-Eastern China related to the high aerosol loadings in autumn when the weather system isrelatively stable? How do aerosols affect autumn precipitation in heavily polluted China? What kinds of precipitation will be affected mostly by aerosol? In this study, we try to address these questions from the perspective of precipitation formation mechanism over Mid-Eastern China which is featured by the heavy pollution, dense meteorological station and records, and frequent precipitation events records [23]. The paper is organized as follows: Data and methodology are described in sections 2. The part of effects of aerosols on autumn precipitation over Mid-Eastern China is presented in section 3. Conclusions and discussions are presented in section 4.2 DATA AND METHODOLOGYThe following types of data are used in this study: (1) Daily observational data were obtained from China Meteorological Data Sharing Service System (/home.do). We screened all daily precipitation records from 1959 to 2008 over 503 observation stations and included them in our following analysis. It also should be noted that the drizzle being not recorded by precipitation instruments was used and assigned the value of 0 mm and only the liquid precipitation was considered in our study.(2) Visibility data were widely used to study the relationship between aerosol and precipitation, due to its relatively long historic records [24-26]. The variability of atmospheric visibility, which is a good measure of environmental pollution, is consistent with that of aerosol optical depth (AOD)[27]. The unit of visibility dataset changed from level (bins) to kilometer after 1980 in China, resulting in systematic discrepancies in data processing. Thus, only the visibility data after 1980 were used in this study and data was further revised by applying the Rosenfeld method [28] to reduce the effects induced by relative humidity and precipitation.(3) We collected the sounding data from Chinese international exchange stations, including the mean monthly air pressure, altitude, temperature, dew temperature and wind speed from January 1951. The dew temperature and air temperature from 28 observational stations were used to calculate the specific humidity, of which only the results under 300 hPa were considered for deducing the perceptible water vapor.(4) Aerosol optical depth, cloud fraction and cloud particle effective radius from Moderate Resolution Imaging Spectroradiometer (MODIS)[29, 30] were used in this study. The AOD at 550 nm as a proxy of aerosol loading was sorted into 10 bins at a regular interval of 0.1. Cloud microphysical and optical parameters for cloud optical depth are sorted244 Journal of Tropical Meteorology Vol.20244into individual AOD bins, of which mean values and standard errors (i.e., / (n 1)1 / 2, where and n are standard deviation and the number of data points, respectively) are then calculated. 3 RESULTS3.1 Change trends of precipitationFigure 1 shows the spatial distributions of trends for seasonal precipitation amounts during the past 50 years over Mid-Eastern China, estimated using the least squares technique. Decreasing trends of spring precipitation were mainly centered in the regions around the Yangtze River and Southeast China, with the largest decreasing trends (i.e. from –2%/decade to –8%/decade) over the Loess Plateau region. Increasing trends of spring mean precipitation were mainly found over the Northeastern China, Beijing, Tianjin, North China Plain, Yunnan-Guizhou Plateau and Tibet Plateau. The decreasing trends for summer precipitation are larger (i.e. from –1%/decade to –5%/decade) than for spring over Northeast China, Beijing, Tianjin, Shandong Peninsula and Loess Plateau. Positive change trends of summer mean precipitation were found over Southern China, with the largest increasing trends (from 1%/decade to 10%/decade) over the Yangtze River Plain. The trends for winter mean precipitation were generally positive over China, except for Beijing, Tianjin and Inner Mongolia. The decreasing trends of precipitation in autumn were distributed more broadly and homogeneously than that in other seasons. Significant decreasing trends of autumn mean precipitation were found over the Yangtze River Plain, Sichuan Plateau, North China Plain and Yunnan-Guizhou Plateau. As a whole, lower amount of precipitation was generated over the Mid-Eastern China, especially over Northeast China, Central China and South China.Figure 1. Spatial distribution of the trend (% per decade) of precipitation in (a) spring (b) summer (c) autumn (d) winter precipitation amount from 1959 to 2008.Figure 2 shows the temporal variation of seasonal mean precipitation averaged for all observationalNo.3 CHEN Si-yu ( ), HUANG Jian-ping ( ) et al. 245245stations in the Mid-Eastern China. Increasing trends of precipitation were found for summer and winter whereas decreasing trends were found for spring and autumn. The trend of autumn mean precipitation was more significant over Yangtze-Delta region (i.e. –5.6%/decade) since 1980 and later on, contributed to the characteristic of autumn mean precipitation variations over Mid-Eastern China.Figure 2. Time series of precipitation anomaly (%) in spring, summer, autumn and winter from 1959 to 2008 averaged over Mid-Eastern China.3.2 Change trends of atmospheric water content Figure 3 shows the temporal variations of autumn atmospheric water content averaged over Mid-Eastern China during 1959–2002. The year of 1975 tends to be the turning point of atmospheric water content, indicating a transition from negative to positive trend. The lowest atmospheric water content during the past 50 years was found at the end of 1960s, while two peaks at the end of 1990s (i.e. 15%) and at the begging of the 21 century (25%) respectively. Most of the regions over Mid-Eastern China experienced a positive change trend of atmospheric water content, especially over Yangtze River, Southeast, Southwest and Northeast of China with an increase of 0.3–0.6 mm/decade generally. The least square method was applied to calculate the change trends of autumn mean precipitation during the past 50 years over Mid-Eastern China. Based on the above analysis, no evidences were found to support that the decreasing autumn precipitation trend over Mid-Eastern China was related to changes of the large-scale atmospheric water content. We believe other factors could play more important roles in influencing the autumn precipitation characteristics over Mid-Eastern China.246Journal of Tropical Meteorology Vol.20246Figure 3. Time series of autumn precipitable water anomaly (%) in autumn from 1959 to 2008 averaged over Mid-Eastern China. The dashed line represents the linear trend of precipitable water anomaly. The solid line represents the moving average of precipitable water.3.3 Effects of aerosols on precipitation3.3.1 S PATIAL VARIATION OF AOD AND VISIBILITYAerosols could directly and indirectly affect the radiation budgets of the earth system, with substantial effects on regional even global climate [31-36]. Thus, further analysis was done to demonstrate the role of aerosols on precipitation over Mid-Eastern China. Fig. 4 shows the spatial distribution of annual mean AOD derived from MODIS retrievals and visibilities from observational data in autumn. AOD values larger than 0.6 were found over the Sichuan Basin, Yellow River, the middle and lower reaches of Yangtze River. The spatial variations of annual mean AOD were also consistent with the findings of previous studies (Luo et al.[37], Wang et al.[38]). Visibility data also showed a consistent spatial pattern with that for AOD. It should be noted that the spatial distribution of visibility was of the opposite sign with that of AOD (i.e. larger AOD with smaller visibility), which gave more confidence to represent the characteristics of aerosol particles using visibility datasets.3.3.2 S VD ANALYSIS OF VISIBILITY AND PRECIPITATIONThe Singular Value Decomposition (SVD) analysis, a useful tool in demonstrating the spatial correlation of two atmospheric variables, has been widely used in various studies [39]. Autumn meanvisibility was treated as the left field whereas the autumn mean precipitation was treated as the right field. The coupling relationship between the anomalous distribution of autumn mean precipitation and the variation of visibility has been investigated using SVD analysis. It should be pointed out that only the simultaneous correlation was considered in this study due to the large spatial variation and short life period of aerosol particles.The variance contribution, cumulative variance and correlation coefficient for the first five SVD modes of singular vector were shown in Table 1. It was found that the first five pairs of singular vector contributed up to 75% of the total variance. Thus, they can be used to depict the coupling relationship of precipitation and visibility variations over Mid-Eastern China. The first mode of visibility and precipitation indicated a significant decrease of temporal coefficient (R =0.87, P <0.01). The heterogeneous correlation coefficient of the right field of the mode for visibility and precipitation was dominated by positive values, with negative correlation found over a few small regions. High positive correlation was found over Southeastern China and Northeastern China (17.5°–36°N, 105°–120°E), which was characterized by the significant coupling of the first mode. Thus, it can be inferred from the consistence of spatial variations between aerosol and precipitation that aerosol couldNo.3 CHEN Si-yu ( ), HUANG Jian-ping ( ) et al. 247247have large impacts on precipitation, with more aerosolloadings and lower precipitation.Figure 4. Spatial distribution of AOD retrievals from MODIS averaged for the autumns of 2002-2008 (a) and the corrected visibility for the autumns of 1959-2008 (b) over Mid-Eastern China.Table 1. The variance contribution, cumulative variance and correlation coefficient explained by the first five pairs (q =1, 2, 3, 4, 5) of singular vector in this study.Singular vector Variance contribution Cumulativevariance Correlation coefficient q =1 36.56% 36.56% 0.83 q =2 21.34% 58.19% 0.90 q =3 7.94% 66.13% 0.92 q =4 6.50% 72.63% 0.87 q =5 4.86%77.47%0.90By absorbing and scattering radiation, aerosols could alter regional atmospheric stability and vertical motions, and affect the large-scale circulation and hydrologic cycle with significant regional climate effects [40-43]. In addition, aerosol particles serve ascondensation nuclei for the formation of both cloud droplets and atmospheric ice particles, exerting large influence on the formation of precipitation [44-48]. With the rapid development of urbanization, pollutant emission has increased dramatically over Mid-Eastern China for the last few decades. Due to the production of more black carbon aerosols and sulfate aerosols, Mid-Eastern China became the unique experimental region for studying the impacts of aerosol on regional climate and hydrological cycle. Next, we try to focus on the effects of aerosol mechanisms on autumn precipitation from the perspective of atmospheric stability conditions and the cloud microphysical conditions.3.3.3 C HANGE TRENDS OF CONVECTIVE AVAILABLEPOTENTIAL ENERGY (CAPE), CONVECTIVE INHIBITION ENERGY AND REVISED VISIBILITYFigure 5 shows the temporal variations of CAPE, Convective Inhibition Energy (CIN) and revised visibility in autumn averaged over Mid-Eastern China during the past 50 years. High CAPE was accompanied by low CIN, suggesting an inverse correlation between CAPE and CIN. CIN increased by 28.67 J/kg per decade, while CAPE decreased by 12.81 J/Kg per decade. More specifically, air stability increased significantly after the 1980s, accompanied by the decrease of CIN by –2.1%/year and the increase of CAPE by 4.7%/year.A decreasing trend (i.e. –2.7%/year) was found for visibility variation, which was consistent with the trend of CAPE. Thus, it can be concluded that aerosols could suppress vertical motion of air and increase regional atmospheric stability, resulting in the decrease of autumn mean precipitation.3.3.4 R ELATIONSHIP BETWEEN AEROSOLS AND CLOUDEFFECTIVE PARTICLE RADIUSFigure 6 shows the comparison of autumn mean aerosol optical depth and cloud droplet effective radius under different cloud top temperature and liquid water path for 2002–2008. The solid line represents the relationship between aerosols and cloud effective particle radius when liquid water path exceeds 70 g m -2. A positive correlation between cloud droplet effective radius and liquid water path could be found when the magnitude of aerosol optical depth was lower than 0.2. Cloud droplet effective radius decreases quickly with the increase of aerosol optical depth, which is more significant with higher cloud top temperature. A decrease of cloud droplet effective radius (i.e. 5 m) was found with the increase of aerosol optical depth when cloud droplet effective radius exceeds 289 K. Thus, it can be inferred that more aerosols in autumn over Mid-Eastern China lead to smaller cloud droplet effective radius, which in turn decreases the conversion efficiency of cloud droplet into raindrop248 Journal of Tropical Meteorology Vol.20248and suppresses the formation of precipitation in autumn.Figure 5. The first SVD mode of the autumn visibility and autumn precipitation for the period 1980-2005. (a) The temporal coefficients of autumn visibility (solid line) and autumn precipitation (dash line); (b) The spatial pattern of heterogeneous correlation coefficient.From the perspective of precipitation formation mechanism, increased atmospheric stability and modified cloud microphysics could result in the decrease of autumn precipitation over Mid-Eastern China. Increased atmospheric stability induced by more aerosols may decrease the surface net radiation, inhibit the ascending motion of air and decrease the formation of precipitation. On the other hand, aerosols also change the characteristics of cloud microphysics by influencing the process of cloud condensation nucleus and lead to decreased precipitation. 4 CONCLUSIONS AND DISCUSSION In this study, the effects of aerosol on the autumn precipitation over Mid-Eastern China were investigated using ground observations, satellite retrievals and NCEP reanalysis dataset. Main conclusions are as follows.Precipitation decreased more significantly in autumn (i.e. 54.3 mm/decade) than in other seasons during the past 50 years over Mid-Eastern China. The decreasing trend of autumn precipitation was more significant after the 1980s, with an average decrease of 5.6% per year.From the perspective of precipitation formation mechanisms, water vapor, atmospheric stability and cloud microphysics could lead to the decrease of autumn precipitation. We found that the increased aerosols produced by enhanced industrialization and environmental pollution could result in the change of atmospheric stability and cloud microphysics, which were at least partly responsible for the decreased autumn precipitation observed over Mid-Eastern China for the past 20 years.The effects of aerosol on autumn precipitation were more outstanding than that on other seasons because the weather system in autumn was relatively stable compared to that in other seasons and the dynamical influence was greater than that of thermal dynamical activity. The wet removal of precipitation on aerosol was relatively small in autumn compared to that in summer, leading to the greater effects of aerosol on precipitation in autumn than in summer. Generally speaking, the high frequency of dust aerosol occurred in spring, which may be caused by relatively high frequency of convective weather outbreak and the dry loose soil. However, the impact of dynamical activity in spring also plays an important role in the spring precipitation change. Therefore it is hard to distinguish which factor is the most significant for precipitation change in spring. In winter, various types of precipitation could occur, including rain, snow and hail, leading to larger difficulty in detecting precipitation in winter than in other seasons. Furthermore, relatively more stable weather systems near the surface and the stable visibility could both lead to difficulty in studying the aerosol variations in winter. Thus, the precipitation change in winter was not considered in this study.In summary, the effects of aerosols on autumn precipitation change over Mid-Eastern China were demonstrated using observed datasets from the perspective of precipitation formation mechanisms. More efforts will be spent to study the physical interactions between precipitation change and aerosol variations.No.3 CHEN Si-yu ( ), HUANG Jian-ping ( ) et al. 249249Figure 6. Time series of convective available potential energy (dash line), convective inhibition energy (solid line) and revised visibility (red histogram) anomalies in autumn over Mid-Eastern China. The red line represents linear trend of revised visibility anomaly.Acknowledgement: We appreciate three anonymous reviewers for their valuable comments and suggestions. The contribution of PNNL in this research was supported by the Office of Science of the U.S. Department of Energy as part of the Regional & Global Climate Modeling (RGCM) Program through the bilateral agreement between U.S. Department of Energy and China Ministry of Science and Technology on regional climate research. The Pacific Northwest National Laboratory is operated for DOE by Battelle Memorial Institute under contract DE-AC06-76RLO 1830. And we also thank Dr. SHANG Ke-zheng, Dr. WANG Tian-he, SHAN Hai-xia and WANG Shan-shan from Lanzhou University for their help in this study.REFERENCES:[1] ZHU Qian-gen, LIN Jin-rui, SHOU Shao-wen, et al. The principle and method of Synoptic meteorology [M]. Beijing: China Meteorological Press, 2000: 458.[2] JIAN Mao-qiu, LUO Hui-bang, QIAO Yun-ting. Linkage between the interannual variation patterns of seasonal SST in Indian Ocean-Pacific and their relationship with the summer rainfall over China [J]. J. Trop. Meteor., 2006, 22(2): 131-137. [3] ZHANG Tian-Yu, SUN Zhao-bo, LI Zhong-Xian, et al. Relation between Spring Kuroshto SSTA and summer rainfall in China [J]. J. Trop. Meteor., 2007, 23(2): 189-195.[4] CHEN Shao-dong, WANG Qian-qian, QIAN Yong-pu. Preliminary discussions of basic climatic characteristics of precipitation during raining seasons in regions south of Changjiang River and its relationship with SST anomalies [J]. J. Trop. Meteor., 2003, 19(3): 260-268.[5] JIN Zu-hui, LUO Shao-hua. On the relationship between rainfall anomaly in middle and lower Yangtze valley during the Mei-Yu season and the anomaly of sea-surface temperature in South China Sea [J]. Acta Meteor. Sinica, 1986, 44(3): 360-372.[6] CHEN Yun, SHI Neng. Spatial and temporal distribution of autumn precipitation and temperature in China and climatic change [J]. J. Nanjing Instit. Meteor., 2003, 26(5): 662-630. [7] XU Gui-Yu, LIN Chun-Yu. Survey of the causes and features of autumn rain in Western China [J]. Sci. Meteor. Sinica, 1994, 12(4): 149-154.[8] LI Yao-hui, LI Dong-liang, ZHAO Qing-Yun. A study on spring rainfall anomaly in Northwest China and Pacific SSTA features in autumn and their correlations [J]. Plateau Meteor., 2000, 19(1): 100-110.[9] LI Yao-hui, LI Dong-liang, ZAHO Qing-yun. An analysis on characteristic of autumn rainfall anomaly in Northwest China [J]. Plateau Meteor., 2001, 20(2): 94-99.[10] LI Yao-hui, LI Dong-liang, ZHAO Qing-yun et al. Effect of ENSO on the autumn rainfall anomaly in northwest China [J]. Clim. Environ. Res., 2000, 25(2): 102-112.[11] SONG Lian-chun, ZHANG Cun-jie. Changing features of precipitation over Northwest China during the 20th century [J]. J. Glaciol. Geocry., 2003, 25(2): 143-147.[12] ZHANG Cun-jie, GAO Xue-jie, ZHAO Hong-yan. Impact of global warming on autumn precipitation in Northwest China [J]. J. Glaciol. Geocry., 2003, 25(2): 157-164.[13] SHI Neng. The temporal and spatial characteristics of monthly temperature and rainfall field during autumn and winter in China and their application in early summer precipitation forecasting [J]. Chin. J. Atmos. Sci., 1988, 12(3): 283-291.[14] HUANG J, MINNIS P, LIN B, et al. Possible influences of Asian dust aerosols on cloud properties and radiative forcing observed from MODIS and CERES [J]. Geophys. Res. Lett., 2006, 33, L06824, doi: 10.1029/2005GL024724.[15] CHEN Si-yu, HUANG Jian-ping, LIU Jin-jin, et al. Effects of dust aerosols on cloud in semi-arid regions as inferred from OMI and MODIS retrievals [J]. Adv. Ear. Sci., 2010, 25, doi: 1001-8166(2010) add- 0188-11.[16] HUANG J, MINNIS P, LINin B, et al. Advanced retrievals of multilayered cloud properties using multispectral。
1为提高生产对土壤进行整体调节,农业及生态系统服务
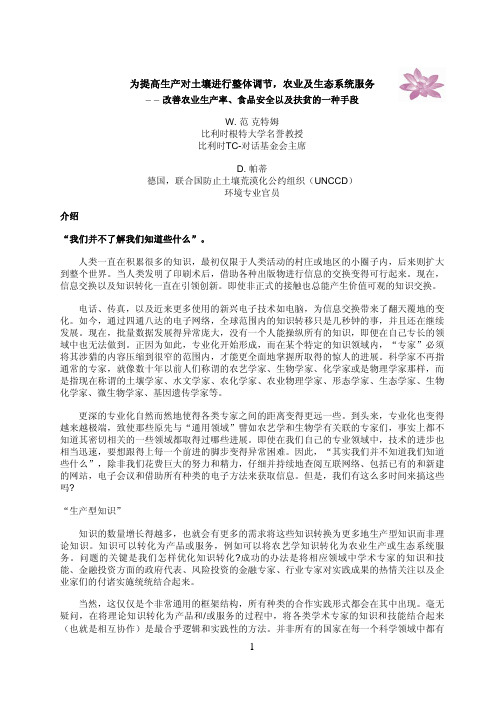
——改善农业生产率、食品安全以及扶贫的一种手段W. 范克特姆比利时根特大学名誉教授比利时TC-对话基金会主席D. 帕蒂德国,联合国防止土壤荒漠化公约组织(UNCCD)环境专业官员介绍“我们并不了解我们知道些什么”。
人类一直在积累很多的知识,最初仅限于人类活动的村庄或地区的小圈子内,后来则扩大到整个世界。
当人类发明了印刷术后,借助各种出版物进行信息的交换变得可行起来。
现在,信息交换以及知识转化一直在引领创新。
即使非正式的接触也总能产生价值可观的知识交换。
电话、传真,以及近来更多使用的新兴电子技术如电脑,为信息交换带来了翻天覆地的变化。
如今,通过四通八达的电子网络,全球范围内的知识转移只是几秒钟的事,并且还在继续发展。
现在,批量数据发展得异常庞大,没有一个人能操纵所有的知识,即便在自己专长的领域中也无法做到。
正因为如此,专业化开始形成,而在某个特定的知识领域内,“专家”必须将其涉猎的内容压缩到很窄的范围内,才能更全面地掌握所取得的惊人的进展。
科学家不再指通常的专家,就像数十年以前人们称谓的农艺学家、生物学家、化学家或是物理学家那样,而是指现在称谓的土壤学家、水文学家、农化学家、农业物理学家、形态学家、生态学家、生物化学家、微生物学家、基因遗传学家等。
更深的专业化自然而然地使得各类专家之间的距离变得更远一些。
到头来,专业化也变得越来越极端,致使那些原先与“通用领域”譬如农艺学和生物学有关联的专家们,事实上都不知道其密切相关的一些领域都取得过哪些进展。
即使在我们自己的专业领域中,技术的进步也相当迅速,要想跟得上每一个前进的脚步变得异常困难。
因此,“其实我们并不知道我们知道些什么”,除非我们花费巨大的努力和精力,仔细并持续地查阅互联网络、包括已有的和新建的网站,电子会议和借助所有种类的电子方法来获取信息。
但是,我们有这么多时间来搞这些吗?“生产型知识”知识的数量增长得越多,也就会有更多的需求将这些知识转换为更多地生产型知识而非理论知识。
Effect of water-filled pore space on carbon dioxide and nitrous oxide production in tilled and non-t

Effects of tillage and soil moisture on forage production and N2O emissions from simulated grazing of a winter forage crop.Steve Thomas, Glyn Francis, Hayley Barlow, Michael H. Beare, Duncan Hedderley, Ruth Butler, Richard N. Gillespie and Frank TableyCrop & Food Research, Private Bag 4704, Christchurch, New Zealand. Email: thomass@ AbstractGrazing of short-term forage crops grown for high quality feed over winter can cause extensive compaction and pugging of soils. This may lead to reduced re-growth of crops and to enhanced N2O emissions. Modification of management practices may reduce these negative effects, for example decreasing the intensity of tillage used in the establishment of forage crops and restricting grazing when soils are wet.We designed a replicated field trial to investigate the effects of simulated cattle grazing at three soil moisture contents (< field capacity, field capacity and > field capacity) on N2O emissions from an autumn sown (March) winter forage crop (triticale) established by three tillage practices: (a) intensive, IT, (b) minimum, MT, or (c) no tillage, NT.Treading wet soil greatly increased (up to 8 times) the amount of N2O emitted. The highest cumulative N2O emissions after 90 days were from IT (14.9 kg N/ha) and MT (12.7 kg N/ha) urine-applied plots treaded at > field capacity. Treading at < field capacity did not affect N2O emissions (1.5 to 2.4 kg N/ha) where urine was applied. Where urine was not applied, N2O emissions were negligible (< 0.5 kg N/ha). Soil compaction reduced dry matter production (June to October) by up to 50 % in IT and MT plots treaded at > field capacity. Dry matter production in the NT plots was unaffected by treading.Key WordsNitrous oxide, tillage, compaction, urine, forageIntroductionThe grazing of winter forage crops by dairy cows on wet soil can result in soil compaction that may limit crop re-growth. Compaction influences a range of soil physical properties, such as bulk density, soil porosity, and water holding capacity, that are important to maintaining plant growth and environmental quality (Lipiec and Hatano 2003). The effects of pastoral grazing on soil physical condition and pasture performance have received some research attention (Drewry et al. 2001; Singleton and Addison 1999). However, the effects of grazing winter forage crops on subsequent dry matter (DM) production are not well understood.In pastoral systems, grazing by livestock can result in the production of large amounts of nitrous oxide (N2O), a potent greenhouse gas, because the high nitrogen concentrations in livestock urine stimulate rapid denitrification, especially when soil moisture contents are high (de Klein and van Logtestijn 1994; Oenema et al. 1997). Water filled porosity has been identified as a key indicator of N2O emissions since moisture content will affect diffusion of oxygen through the soil matrix (Linn and Doran 1984). In general, high N2O emissions from denitrification can occur in a wide range of intensive agricultural systems when water-filled pore space (WFPS) exceeds 60 % (Dobbie and Smith 2003).The effects of soil compaction on N2O emissions have been reported for some intensive agricultural systems (Ball et al. 1999b; Hansen et al. 1993; Ruser et al. 1998), and the effects of compaction from stock treading on N2O emissions suggest that compaction by stock treading could double emissions from pasture (Oenema et al. 1997). In New Zealand, soil compaction caused a threefold increase in N2O emissions from a urine amended dairy pasture (Bhandral et al. 2003).One of the key differences between winter grazing of pasture and forage crops is the use of tillage to establish the latter. Tillage can significantly alter soil structure and thereby increase susceptibility to compaction, especially when soils are wet. While a number of studies have investigated the effects of tillage on soil N2O emissions, there is a need for information on how the interaction of tillage practicesand compaction affects N 2O emissions (Yamulki and Jarvis 2002). Some studies have reported higher N 2O emissions from no-tillage than conventional tillage soils (Ball et al. 1999b), as a result of increased soil moisture content, water conservation and lower soil gas diffusivity, whereas other studies report no significant effects of tillage on N 2O emissions (Elmi et al. 2003; Yamulki and Jarvis 2002).To address some of these uncertainties we conducted a field trial to test the hypothesis that the use of no-tillage practices to establish forage crops out of pasture will reduce soil compaction, lower emissions of N 2O and increase dry matter production following grazing as compared to conventional and minimum tillage systems.Materials and methodsThe field trial was conducted from March to October 2003 at Lincoln, Canterbury. The soil at the site was a Wakanui silt loam classified as a mottled immature pallic soil (Hewitt 1993). A grass/clover pasture (>15 years old) was sprayed with glyphosate prior to sowing (3 March) a multi-grazing triticale crop (cv. Doubletake). The seedbed was prepared with either (a) intensive (IT: plough to 20 cm depth, maxi-till, roll and harrow), (b) minimum (MT: disc to 10 cm depth, roll and harrow) or (c) no-tillage (NT)practices. These main plots were 9 x 9 m in size, replicated three times. Production of DM was estimated from sub-samples cut from each plots in June, after which the site was mowed to 10 cm height. Six split-plots (3 m x 1 m) were then established within each main tillage plot to determine the effects of soil moisture during grazing, animal treading and urine on soil compaction, N 2O emissions and plant performance (Table 1). A buffer strip of at least 1 m surrounded each split plot.Table 1: Split plot treatments imposed at simulated grazingSplit Plot Treatment Soil moisture content Treading Urine application 1 < FC a Yes Yes2 FC Yes Yes3 > FC Yes Yes4 FC Yes No5 FC No Yes6 FC No No aSimulated grazingSoil moisture contents for subplots at or above FC (Table 1) were adjusted by applying spray irrigation immediately before grazing. Soil moisture contents <FC (Split plot Treatment 1, Table 1) were achieved by covering subplots with cloche frames during rainfall events from mid-May until simulated grazing. A single grazing event was simulated in June 2003. Treading was simulated using a mechanical cow hoof that applied a pressure of 220 kPa to the soil surface, representing the treading impact of an adult Friesian cow (Di et al. 2001). The soil was treaded by placing the mechanical hoof on the soil surface, then pneumatically pressing the hoof in to the soil. This procedure was repeated on the adjacent soil surface until the whole plot had been treaded. In addition to treading, synthetic urine (Clough et al. 1998) was uniformly applied by hand at a rate of 800 kg N/ha. Dry matter production following grazing wascalculated by the difference in triticale DM remaining at the time of simulated grazing and DM estimates from sub-samples cut from each subplot at the end of the study.N 2O measurementsN 2O fluxes were determined using a closed chamber technique (Hutchinson and Mosier 1981). Chambers (10 cm depth) were made from 25 cm diameter PVC pipe with welded lids. Bases (15 cm depth), made from the same diameter PVC pipe, were inserted into the soil (5 cm depth) to enable gas fluxes to be measured at the same position within each plot. A water-filled channel at the top of each base produced a gas tight seal with the chamber during measurements. Within each subplot, N 2O concentrations were measured in the chamber headspace at three time intervals (0, 20 and 40 minutes) after chamber closure. Chamber heights were extended during the trial to accommodate the growing triticale. Gas samples were analysed using a gas chromatograph (GC-17A, Shimadzu Corporation, Kyoto) fitted with a 63Ni-Electron capture detector. Measurements were made on 27 occasions over 92 days.Soil measurementsSoil bulk density was measured at a depth of 0-7.5 cm within 3 weeks of the simulated grazing. On eight occasions during the period of gas sampling, soil mineral N was extracted from soil samples (0-25 cm depth) using 2M potassium chloride and analysed using a Rapid Flow Analyser (Astoria-Pacific Inc., Clackamas, Oregon). Volumetric soil moisture content (0-10 cm) was measured hourly in each plot using ECH2O capacitance probes (Decagon Devices Inc., Pullman, Washington) connected to a datalogger and multiplexers (Campbell Scientific Inc., North Logan, Utah). WFPS (0-7.5 cm) was estimated by: WFPS = volumetric moisture content/(1-(soil bulk density/soil particle density)). We have assumed that the volumetric moisture content at 0-7.5 cm was similar to that measured by the capacitance probes between 0-10cm. Rainfall, air temperature and soil temperature were also measured at the site.Statistical analysesThe effects of tillage, treading, soil moisture content at treading and urine on dry matter production following grazing were analysed using split-plot analysis of variance. Contrasts between the various split plots were included in the analysis of variance. Treatment effects on bulk density were analysed using split-split plot analysis of variance. A mixed model fitted using REML (Residual Maximum Likelihood) analysis tested for the effects of tillage, treading, soil moisture content at treading and urine on N2O emissions, WFPS and mineral N with time. The N2O flux and mineral N data required log transformation to make the variance more homogeneous; the results presented have been back transformed. Comparison of the N2O flux and mineral N means between tillage treatments is made using the least significant ratio (LSR). The LSR is the smallest ratio between two back-transformed means (largest mean/smallest mean) such that the larger mean is significantly greater than the smallest mean. For all the statistical analyses a significance level of 5% was used to test for treatment effects. Analyses were performed using the GenStat (version 7) software package.Results and discussionBulk densityPrior to treading in June, soil bulk density and WFPS were similar at 0-7.5 cm depth (range 1.1–1.2g/cm3) for all three tillage systems. At 7.5–25 cm depth, however, bulk density and WFPS were lower under IT than MT or NT (data not shown). Treading at field capacity increased surface soil bulk density (0 to 7.5 cm) in all tillage treatments, the greatest increase occurring under IT (Figure 1). Treading at moisture contents above field capacity (>FC) greatly increased the bulk density and WFPS of surface (0-7.5 cm) soil from IT plots (Figure 1). There was less compaction of surface soil when treading occurred below field capacity (<FC), and bulk density values were similar to the plots that had no treading.Soil mineral N levels and N2O emissionsThroughout the trial, soil mineral N contents at 0-25 cm depth remained above 50 kg N/ha in all plots (Figure 2). The application of urine in June resulted in a rapid increase in soil mineral N contents to 400 kg N/ha, which then decreased steadily during the remaining 10 weeks of the trial. There were significant effects (P < 0.05) of tillage, moisture content at treading, urine and treading treatments on mineral N (0-25 cm). Mineral N significantly changed over time and there were additional significant interactions between the moisture content at treading and time, and urine and time.There were significant effects of treading, moisture content at treading and urine (P < 0.001) and tillage method on N2O emissions (P < 0.05). Overall, the greatest cumulative N2O emission was from the IT soil and lowest from the NT soil (Table 2). The highest hourly fluxes of N2O were associated with high soil moisture and WFPS (Figure 2), following rainfall events, suggesting that the N2O emissions are largely due to denitrification, as reported in other studies (de Klein and van Logtestijn 1996; Linn and Doran 1984). The highest mean N2O fluxes and greatest cumulative N2O emissions were from intensively tilled plots, compacted at moisture contents >FC (Figure 2 and Table 2). The highest mean N2O fluxes from the IT and MT plots, treaded at >FC with urine applied, were higher than those measured from pasture plots on a moderately drained silt loam in Canterbury (0.7 mg N/m2/h) that had not been treaded, but had urine applied at a rate of 655 kg N/ha (de Klein et al. 2003). While, the fluxes from our study are lower than those measured from pasture on a poorly drained silt loam in Otago, (4.9 mg N/m2/h) that had urine applied (592 kg N/ha), but had not been treaded (de Klein et al. 2003).and untreaded plots at FC (hatched bar) following IT, MT and NT. Data are means of three replicates. The error bar represents the LSD (P < 0.05) for comparisons of the tillage and moisture treatments that were treaded.2compacted when soil was above field capacity and urine had been applied to plots (n = 3) growing triticale following three tillage methods. N2O and mineral N data are back-transformed from log-transformed data. For comparisons of N2O flux between different tillage treatments the LSR (P < 0.05) = 2.26. For comparison of mineral N between different tillage treatments the LSR (P < 0.05) = 1.9.N 2O emissions from compacted soils were up to eight times greater than those of untreaded soil (Table 2). Increased N 2O production due to soil compaction has been observed in several pastoral land-use and other intensive agricultural systems (e.g. Ball et al. 1999b; Ruser et al. 1998; Yamulki and Jarvis 2002). Bhandral et al. (2003) measured a three-fold increase in cumulative N 2O emissions between tractor-compacted (9.2 kg N/ha) and uncompacted (2.9 kg N/ha) urine amended pasture plots on a Manawatu fine sandy loam soil. In contrast to a number of other studies, we found no significant difference in N 2O emissions between the NT and IT plots that had not been compacted (Table 2). These N 2O emissions were small compared to those from plots that had been compacted when the soil was at or above FC (Table 2).WFPS and mineral N contents were not good predictors of the amount of N 2O emitted as has been shown elsewhere (Ball et al. 1999b; Yamulki and Jarvis 2002). From their study of short-term effects of tillage and compaction, Yamulki and Jarvis (2002) suggested that other factors affecting the production, transport and residence time of N 2O were responsible for differences in N 2O emissions. The key soil properties affected by compaction that may influence the production and transport of N 2O and rate of oxygen diffusion through the soil matrix include macroporosity, soil pore tortuosity and water holding capacity (Ball et al. 1999a).Table 2. Cumulative N 2O emissions for a 90-day period following grazing of intensive, minimum or no tillage triticale plots. Data are means of three replicates.Split plot treatments Main plotCumulative N 2O Flux (kg N/ha)Moisture contentTreading Urine addedIT MT NT <FC Yes Yes 1.45 1.87 2.04>FC Yes Yes 14.86 12.68 4.97FC Yes Yes 5.73 3.18 3.0FC No Yes 2.36 1.66 2.07FC Yes No 0.31 0.53 0.37FC No No 0.24 0.28 0.46Urine application had a strong effect on N 2O emissions in this study. Where urine was not applied, cumulative N 2O emissions were very low (Table 2). In contrast, where urine was applied, N 2O emissions were low during the first month following urine application, as soil mineral N contents were also low during this time, but tended to be higher from August onwards, by which time much of the applied urine had been converted to nitrate (Figure 2). From mid-July onwards, emission peaks were recorded following most rainfall events (Figure 2) and corresponded with an increase in the WFPS of the soil.Effects on DM productionDry matter production, before grazing in June, was unaffected by tillage treatment (2400 kg DM/ha). Treading, moisture content at treading and urine application affected the triticale DM production (Figure 3, P < 0.05). There was also some evidence to suggest that tillage method was also important (P = 0.06 for tillage x split plots interaction effect ). As the soil moisture content at treading increased from <FC to >FC, DM production decreased two fold (Figure 3). In contrast, DM production on the NT plots was unaffected by soil moisture content at grazing. The application of urine increased triticale re-growth by up to 50%, this effect being greatest in the NT plots.grazing at 3 moisture contents (< FC, FC and > FC) established by IT, MT or NT (n = 3). The error bar represents the LSD (P < 0.05) for comparison of tillage and moisture treatments.ConclusionsThe use of no-tillage practices to establish a multi-grazing winter forage crop (ex-pasture) had significant benefits over other tillage practices in terms of environmental impacts and forage crop performance. Soil compaction, due to grazing under wet conditions, increased N2O production and decreased forage crop production. Conventional tillage practices (IT) for establishing winter forage are more likely to result in soil compaction than reduced tillage systems. Hence where these winter forages are grazed, establishment by direct drilling is likely to be an important option for mitigating N2O emissions, while maintaining high dry matter yields. Restricting grazing when soils are wet will reduce the risk of increasing N2O emissions and loss of dry matter production.AcknowledgementsThis study was funded by the Foundation for Research Science & Technology under contracts C02X0218 and C02X0304. We wish to thank Lincoln University for the use of their mechanical hoof. ReferencesBall BC, Parker JP, Scott A (1999a) Soil and residue management effects on cropping conditions and nitrous oxide fluxes under controlled traffic in Scotland 2. Nitrous oxide, soil N status and weather.Soil & Tillage Research52, 191-201.Ball BC, Scott A, Parker JP (1999b) Field N2O, CO2 and CH4 fluxes in relation to tillage, compaction and soil quality in Scotland. Soil & Tillage Research53, 29-39.Bhandral R, Saggar S, Bolan NS, Hedley MJ (2003) Nitrous oxide fluxes in soil as influenced by compaction. Proceedings of the New Zealand Grassland Association65, 265-271.Clough TJ, Ledgard SF, Sprosen MS, Kear MJ (1998) Fate of 15N labelled urine on four soil types. Plant and Soil199, 195-203.de Klein CAM, Barton L, Sherlock RR, Li Z, Littlejohn RP (2003) Estimating a nitrous oxide emission factor for animal urine from some New Zealand pastoral soils. Australian Journal of Soil Research41, 381-399.de Klein CAM, van Logtestijn RSP (1994) Denitrification and N2O emission from urine-affected grassland soil. Plant and Soil163, 235-241.de Klein CAM, van Logtestijn RSP (1996) Denitrification in grassland soils in the Netherlands in relation to irrigation, N-application rate, soil water content and soil temperature. Soil Biology and Biochemistry 28, 231-237.Di H, Cameron KC, Milne J, Drewery JJ, Smith NP, Hendry T, Moore S, Reijnen B (2001) A mechanical hoof for simulating animal treading under controlled conditions. New Zealand Journal of Agricultural Research44, 111-116.Dobbie KE, Smith KA (2003) Nitrous oxide emission factors for agricultural soils in Great Britain: the impact of soil water-filled pore space and other controlling variables. Global Change Biology9, 204-218.Drewry JJ, Cameron KC, Buchan GD (2001) Effect of simulated dairy cow treading on soil physical properties and ryegrass pasture yield. New Zealand Journal of Agricultural Research44, 181-190. Elmi AA, Madramootoo C, Hamel C, Liu A (2003) Denitrification and nitrous oxide to nitrous oxide plus dinitrogen ratios in the soil profile under three tillage systems. Biology and Fertility of Soils38, 340-348.Hansen S, Maehlum JE, Bakken LR (1993) N2O and CH4 fluxes in soil influenced by fertilization and tractor traffic. Soil Biology and Biochemistry25, 621-630.Hewitt AE (1993) 'New Zealand Soil Classification.' (Manaaki Whenua - Landcare Research New Zealand Ltd.: Lincoln, New Zealand)Hutchinson GL, Mosier AR (1981) Improved soil cover method for field measurement of nitrous oxide fluxes. Soil Science Society of America Journal45, 311-316.Linn DM, Doran JW (1984) Effect of water-filled pore space on carbon dioxide and nitrous oxide production in tilled and non-tilled soils. Soil Science Society of America Journal48, 1267-1272. Lipiec J, Hatano R (2003) Quantification of compaction effects on soil physical properties and crop growth. Geoderma116, 107-136.Oenema O, Velthof GL, Yamulki S, Jarvis SC (1997) Nitrous oxide emissions from grazed grassland.Soil Use and Management13, 288-295.Ruser R, Flessa H, Schilling R, Steindl H, Beese F (1998) Soil compaction and fertilization effects on nitrous oxide and methane fluxes in potato fields. Soil Science Society of America Journal62, 1587-1595.Singleton PL, Addison B (1999) Effects of cattle treading on physical properties of three soils used for dairy farming in the Waikato, North Island, New Zealand. Australian Journal of Soil Research37, 891-902.Yamulki S, Jarvis SC (2002) Short-term effects of tillage and compaction on nitrous oxide, nitric oxide, nitrogen dioxide, methane and carbon dioxide fluxes from grassland. Biology and Fertility of Soils36, 224-231.。
Effects of afforestation on soil organic carbon and other soil properties土壤文章中文翻译

3. Material and methods
3.1 样点介绍
Site characteristics Location Altitude (m) Topography Black pine Hıdırlık 1235 Downslope Cedar Hıdırlık 1235 Downslope Bare land Hıdırlık 1235 Downslope
9.54 ± 0.95
10.48 ± 0.95 10.01 ± 1.03A 41.69 ± 3.40A
8.25 ± 0.83
8.41 ± 2.01 8.34 ± 1.49 B 30.24 ± 2.98 Ba
Total porosity (TP) %
10–20
Total 0–10 10–20 Total
1. Abstract
目的:这个研究主要是探究在土耳其阿卡亚地区半干旱土地,为了保持水土而 种植的南欧黑松和黎巴嫩雪松,15年后对土壤有机碳和其他土壤性质的影响; 实验方法:土壤样本取自3种土地利用类型,(黑松和雪松和裸地)两种土壤 深度,重复3次取样;测量指标包括土壤有机碳,容重,比重,土壤持水量, 和土壤空隙度。
Aspect
Slope % Parent material Plantation year Mean height of saplings (m)
NW
5–10 Marble 1998 3.11
NW
5–10 Marble 1998 3.72
NW
5–10 Marble – –
Mean diameter of saplings (cm)
Soil depth
4. Results
Soil properties Soil depth(cm) 0–10 10–20 Total Bulk density (BD) g/cm3 0–10 10–20 Total 0–10 10–20 Total 0–10 Clay % 10–20 Total 0–10 Sand % 10–20 Land use types Black pine 1.19 ± 1.74Aa 0.99 ± 1.82b 1.09 ± 1.75A 1.53 ± 0.11A 1.65 ± 0.09A 1.59 ± 0.11A 2.69 ± 0.13A 2.68 ± 0.18 2.69 ± 0.15A 6.88 ± 1.09 7.56 ± 0.87 7.24 ± 1.03 81.63 ± 2.46 81.63 ± 2.46 Cedar 1.49 ± 1.82Aa 0.82 ± 2.54b 1.13 ± 2.34A 1.58 ± 0.03A 1.51 ± 0.09B 1.55 ± 0.07A 2.73 ± 0.21A 2.69 ± 0.19 2.71 ± 0.20A 9.60 ± 2.29 7.87 ± 4.47 8.72 ± 3.56 77.91 ± 2.06 81.88 ± 5.04 Bare land 0.64 ± 1.69 Ba 0.44 ± 1.52b 0.54 ± 1.61 B 1.75 ± 0.09B 1.64 ± 0.11A 1.70 ± 0.11 B 2.52 ± 0.20B 2.62 ± 0.78 2.57 ± 0.01B 8.85 ± 1.34 7.84 ± 5.57 8.22 ± 3.26 77.17 ± 1.61 82.08 ± 1.23
the_effect_of_rubber_particle_size_on_toughening_behaviour_of_rubber-modified_pmma

The objective of this paper is to clarify the effect of the rubber particle size on the toughening behaviour of rubbertoughened PMMA under different fracture test methods, i.e. the impact test and the three- point bending test. For this purpose, a systematic model study has been carried out using core-shell type particles, which are made up of a poly(n-butyl acrylate) (PBA) core and a PMMA outer shell with a uniform particle size and composition. The fracture surfaces and the deformation region were observed by using various microscopy techniques. The deformation mechanism is also discussed in connection with the test method. EXPERIMENTAL
(Keywords: poly(methyl methacrylate); toughening behaviour; particle size)
INTRODUCTION It is well known that poly(methyl methacrylate) (PMMA) can be toughened by the addition of rubbery particles. The deformation and fracture behaviour of rubber-toughened PMMA has been the subject of much study ~-~l. Although many studies have been reported on the mechanical behaviour of rubber-toughened PMMA, the deformation mechanism for rubber-toughened PMMA is still ambiguous, and contradictory results have been reported. PMMA itself is deformed mainly by crazing t2-14, but the deformation mechanism of rubber- toughened PMMA is influenced by the strain rate, the specimen geometry and the test method. Different deformation mechanisms, i.e. shear yielding ~'2'4 and crazing 3"6'9, have been proposed according to the different test methods. Therefore, the deformation of rubber-toughened PMMA may possibly show different behaviour as the test conditions are changed. The effect of the rubber phase fraction on the toughening behaviour is still unclear. Some authors have reported that the fracture toughness (Klc) showed a sharp transition from 7 and brittle to ductile with increasing rubber phase content, others have found that the Ktc values increased monotonically with rubber content to a maximum value and then decreased with further increase of the rubber phase fraction8. With respect to the effect of the particle size, the optimum panicle size for maximum toughness of rubber-toughened PMMA is known to be around
土壤含水量和光照对沙地柏光合生理指标的影响

第41卷 第1期 生 态 科 学 41(1): 77–832022年1月 Ecological Science Jan. 2022收稿日期: 2020-01-03; 修订日期: 2020-02-18 基金项目: 国家重点研发计划(2018YFC0406604)作者简介: 崔英(1993—), 女, 青海湟源人, 硕士研究生, 从事森林培育和林木遗传育种方向,E-mail:******************通信作者: 王占林, 男, 青海贵德人, 研究员, 硕士研究生导师, 主要从事森林培育及经济林木遗传育种方面的研究,E-mail:*****************崔英, 王占林, 张得芳, 等. 土壤含水量和光照对沙地柏光合生理指标的影响[J]. 生态科学, 2022, 41(1): 77–83.CUI Ying, WANG Zhanlin, ZHANG Defang, et al. Effects of soil water content and light on photosynthetic characteristics of Sabina vulgari Ant .[J]. Ecological Science, 2022, 41(1): 77–83.土壤含水量和光照对沙地柏光合生理指标的影响崔英1,2,3, 王占林1,2,3,*, 张得芳1,2,3, 樊光辉1,2,31.青海大学, 西宁 8100162.青海省农林科学院, 西宁 8100163.青海高原林木遗传育种重点实验室, 西宁 810016【摘要】试验采用人工控制土壤水分的方法, 测定不同土壤含水量条件及有效辐射强度下沙地柏苗木的蒸腾速率、净光合速率、水分利用效率等指标, 分析沙地柏在不同土壤含水量和光照条件下影响的光合生理响应特征及其变化规律。
土壤含水量(SWC)用称重法测定, 将硬塑盆中的土壤水分含量设置为7.5%,10%,12.5%,15%,17.5%, 20% 等6个不同的处理, 每天进行称重, 瞬时土壤水分含量用TDR100土壤水分测速仪(上海赛弗生物公司)测定, 用Li-6400光合测定仪(美国LI-COR 公司)测定沙地柏在不同土壤水分条件下光合生理指标。
水肥调控对设施土壤硝态氮含量和含水率的影响

水肥调控对设施土壤硝态氮含量和含水率的影响包雪莲张智勇*周祎郭方亮杨柳青李雅剑叶英杰刘琦韩新奇董志朋(通辽市农牧科学研究所,内蒙古通辽028000)摘要本文以番茄为研究对象,采用灌水下限土壤水吸力和施氮量2因素3水平设计,开展了水肥调控对设施土壤硝态氮含量和含水率的影响研究。
结果表明,土壤硝态氮含量、含水率的动态变化与灌水下限土壤水吸力、施氮量密切相关。
土壤硝态氮含量随着施氮量的增大而增大,两者成正相关关系;土壤含水率随着施氮量的增大而不断变大,两者成正相关关系。
施氮量对硝态氮含量的影响大于灌水下限土壤水吸力对硝态氮含量的影响,而灌水下限土壤水吸力对土壤含水率的影响大于施氮量对土壤含水率的影响。
关键词番茄;水肥调控;灌水下限土壤水吸力;施氮量;设施土壤;硝态氮含量;土壤含水率中图分类号S641.2文献标识码A文章编号1007-5739(2023)17-0164-04DOI :10.3969/j.issn.1007-5739.2023.17.042开放科学(资源服务)标识码(OSID ):Effects of Water and Fertilizer Regulation on Nitrate Nitrogen Content and Moisture Content in Facility SoilBAO XuelianZHANG Zhiyong *ZHOU YiGUO FangliangYANG LiuqingLI YajianYE YingjieLIU QiHAN XinqiDONG Zhipeng(Tongliao Institute of Agriculture and Animal Husbandry,Tongliao Inner Mongolia 028000)AbstractTaking tomatoes as the research object,this paper adopted a 2-factor and 3-level design of irrigationlower limit of soil water suction and nitrogen application rate,studied the effect of water and fertilizer regulation on the nitrate nitrogen content and moisture content of facility soil.The results showed that the dynamic changes in soil nitrate nitrogen content and moisture content were closely related to irrigation lower limit of soil water suction and nitrogen application rate.The nitrate nitrogen content in soil increased with the increase of nitrogen application rate,and therewas a positive correlation between these two;the soil moisture content continuously increased with the increase of nitrogen application rate,and there was a positive correlation between these two.The impact of nitrogen application rate on nitrate nitrogen content was greater than that of irrigation lower limit of soil water suction on nitrate nitrogen content,while the impact of irrigation lower limit of soil water suction on soil moisture content was greater than that of nitrogen application rate on soil moisture content.Keywordstomato;water and fertilizer regulation;irrigation lower limit of soil water suction;nitrogen applicationrate;facility soil;nitrate nitrogen content;soil moisture content基金项目国家土壤质量观测实验站项目(NAES064SQ22)。
水对红薯的影响英语作文
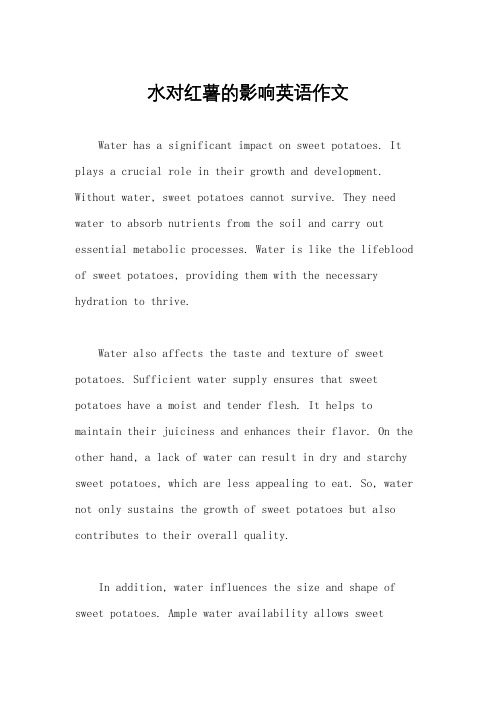
水对红薯的影响英语作文Water has a significant impact on sweet potatoes. It plays a crucial role in their growth and development. Without water, sweet potatoes cannot survive. They need water to absorb nutrients from the soil and carry out essential metabolic processes. Water is like the lifeblood of sweet potatoes, providing them with the necessary hydration to thrive.Water also affects the taste and texture of sweet potatoes. Sufficient water supply ensures that sweet potatoes have a moist and tender flesh. It helps to maintain their juiciness and enhances their flavor. On the other hand, a lack of water can result in dry and starchy sweet potatoes, which are less appealing to eat. So, water not only sustains the growth of sweet potatoes but also contributes to their overall quality.In addition, water influences the size and shape of sweet potatoes. Ample water availability allows sweetpotatoes to grow larger and plumper. It helps them to absorb more nutrients and expand in size. Conversely, water scarcity can stunt their growth and lead to smaller and misshapen sweet potatoes. Therefore, water is essential for the development of sweet potatoes, determining their size and appearance.Moreover, water is crucial in preventing diseases and pests in sweet potatoes. Adequate watering helps to maintain the health of sweet potato plants, making them less susceptible to diseases and pests. It prevents thesoil from becoming dry and creates an unfavorable environment for pathogens and insects. So, water acts as a protective shield for sweet potatoes, safeguarding them from potential threats.Furthermore, water is essential for the storage and preservation of sweet potatoes. Sweet potatoes need to be stored in a cool and humid environment to maintain their freshness and prevent them from drying out. Water can help to create the ideal storage conditions by providing the necessary moisture. It ensures that sweet potatoes stayfirm and retain their nutritional value for a longer duration.In conclusion, water has a profound impact on sweet potatoes. It is indispensable for their growth, taste, size, disease prevention, and storage. Without water, sweet potatoes would not be able to thrive and reach their full potential. So, let's appreciate the significance of waterin the cultivation and enjoyment of sweet potatoes.。
Cultivation effects on soil biological properties, microfauna
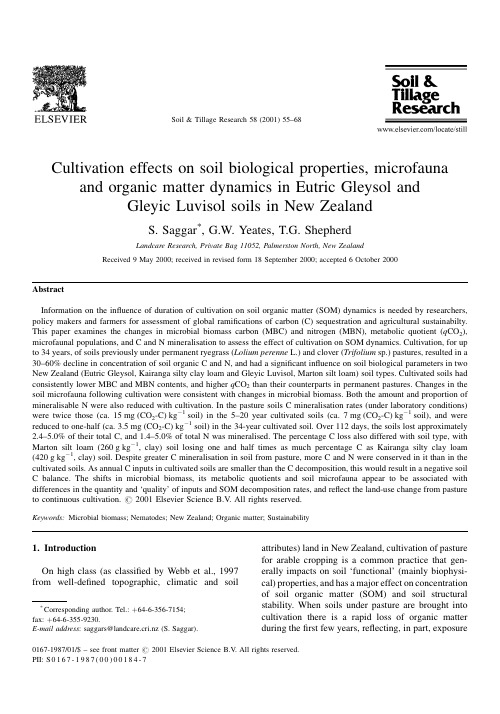
Cultivation effects on soil biological properties,microfaunaand organic matter dynamics in Eutric Gleysol andGleyic Luvisol soils in New ZealandS.Saggar *,G.W.Yeates,T.G.ShepherdLandcare Research,Private Bag 11052,Palmerston North,New ZealandReceived 9May 2000;received in revised form 18September 2000;accepted 6October 2000AbstractInformation on the in¯uence of duration of cultivation on soil organic matter (SOM)dynamics is needed by researchers,policy makers and farmers for assessment of global rami®cations of carbon (C)sequestration and agricultural sustainabilty.This paper examines the changes in microbial biomass carbon (MBC)and nitrogen (MBN),metabolic quotient (q CO 2),microfaunal populations,and C and N mineralisation to assess the effect of cultivation on SOM dynamics.Cultivation,for up to 34years,of soils previously under permanent ryegrass (Lolium perenne L.)and clover (Trifolium sp.)pastures,resulted in a 30±60%decline in concentration of soil organic C and N,and had a signi®cant in¯uence on soil biological parameters in two New Zealand (Eutric Gleysol,Kairanga silty clay loam and Gleyic Luvisol,Marton silt loam)soil types.Cultivated soils had consistently lower MBC and MBN contents,and higher q CO 2than their counterparts in permanent pastures.Changes in the soil microfauna following cultivation were consistent with changes in microbial biomass.Both the amount and proportion of mineralisable N were also reduced with cultivation.In the pasture soils C mineralisation rates (under laboratory conditions)were twice those (ca.15mg (CO 2-C)kg À1soil)in the 5±20year cultivated soils (ca.7mg (CO 2-C)kg À1soil),and were reduced to one-half (ca.3.5mg (CO 2-C)kg À1soil)in the 34-year cultivated soil.Over 112days,the soils lost approximately 2.4±5.0%of their total C,and 1.4±5.0%of total N was mineralised.The percentage C loss also differed with soil type,with Marton silt loam (260g kg À1,clay)soil losing one and half times as much percentage C as Kairanga silty clay loam (420g kg À1,clay)soil.Despite greater C mineralisation in soil from pasture,more C and N were conserved in it than in the cultivated soils.As annual C inputs in cultivated soils are smaller than the C decomposition,this would result in a negative soil C balance.The shifts in microbial biomass,its metabolic quotients and soil microfauna appear to be associated with differences in the quantity and `quality'of inputs and SOM decomposition rates,and re¯ect the land-use change from pasture to continuous cultivation.#2001Elsevier Science B.V .All rights reserved.Keywords:Microbial biomass;Nematodes;New Zealand;Organic matter;Sustainability1.IntroductionOn high class (as classi®ed by Webb et al.,1997from well-de®ned topographic,climatic and soilattributes)land in New Zealand,cultivation of pasture for arable cropping is a common practice that gen-erally impacts on soil `functional'(mainly biophysi-cal)properties,and has a major effect on concentration of soil organic matter (SOM)and soil structural stability.When soils under pasture are brought into cultivation there is a rapid loss of organic matter during the ®rst few years,re¯ecting,in part,exposureSoil &Tillage Research 58(2001)55±68*Corresponding author.Tel.: 64-6-356-7154;fax: 64-6-355-9230.E-mail address :saggars@ (S.Saggar).0167-1987/01/$±see front matter #2001Elsevier Science B.V .All rights reserved.PII:S 0167-1987(00)00184-7of more surfaces due to soil disturbance and dry matter removed during harvesting(Sparling et al.,1992; Shepherd et al.,2001).Soil organic matter is important because of its in¯uence on soil physical,chemical and biological properties and processes.It helps to create a favourable medium for physical processes,chemical reactions,and biological activity.The multi-faceted role of SOM must therefore be taken into considera-tion in any assessment of`soil quality'and`sustain-able land management'.Low concentration of organic matter can have a deleterious effect on offsite envi-ronment because it is often associated with decreased soil fertility,water holding capacity and water in®l-tration,and increased erosion.Decreases in SOM also have global environmental rami®cations for C seques-tration and net emission of greenhouse gases.Previous studies indicate that intensive cropping in the North Island,New Zealand,may give rise to a number of deleterious effects that greatly in¯uence the sustain-ability of grain production systems.The extent of deleterious effects may depend on the initial concen-tration of organic matter,the amount and type of clay minerals in the soil,and both years of cultivation and its seasonal timing.Quantitative information is needed on the changes in concentration of SOM,and on the rate of its decomposition to assess the in¯uence of cultivation on`SOM sustainability'in particular,and on soil quality in general.This information is also needed for future projections of changes in soil C budgets.Recently there has been increased interest in the importance of biological properties as indicators of changes in soil quality(e.g.,Turco et al.,1994).Soil biological properties have the potential to be early and sensitive indicators of soil stress or productivity changes,and there is considerable evidence they can be used to evaluate the in¯uence of management and land-use on soils(Yeates et al.,1998;Saggar et al., 1999).Further,SOM turnover controls the¯uxes of nutrients.Microbial biomass measurements combined with soil respiration(metabolic quotient,q CO2)have frequently been used as an index of soil development or degradation(Insam and Domsch,1988)and to assess the quality of organic matter input(Anderson and Domsch,1990).Interactions between soil micro-organisms and soil microfauna,particularly Protozoa and Nematoda,largely control SOM turnover(Elliot et al.,1984).Earlier studies in New Zealand have indicated a marked loss of concentration of soil organic C and microbial C with continuous cultivation in soils in the Manawatu(Hart et al.,1988;Sparling et al.,1992; Aslam et al.,1999).Shepherd et al.(2001)studied the effect of cultivation on soil organic C,functional chemical composition of SOM,and soil structure in soils of contrasting mineralogy.They found that soil susceptibility to structural degradation increased with years of cultivation,and from light textured to heavier textured soils.Because cultivation causes profound changes in the soil physical and chemical properties, and populations of microfauna and macrofauna (Yeates et al.,1998;Aslam et al.,1999;Shepherd et al.,2001),it is relevant to quantify its effects on soil microbial and microfaunal populations and on SOM dynamics.Effect of land-use change and duration of cultivation on global rami®cations of carbon(C) sequestration and agricultural sustainabilty are major issues for researchers,policy makers and farmers.This work was designed to test how the change in land-use from pasture to cropping and the duration of cropping affect SOM dynamics.Such information may help to interpret the impact of cultivation on SOM dynamics and assess its sustainability under various conditions. Our objectives were to determine changes in soil biological properties,microfauna and SOM dynamics from short-,medium-and long-term cropped and adjacent pasture soils with known histories of manage-ment.We used laboratory incubations to determine the relative C turnover rates and estimated the C inputs,to identify C losses.2.Materials and methods2.1.SitesSites used in this study represent regularly fertilised grazed pastures,sown with introduced grasses(Lolium perenne L.)and legumes(Trifolium sp.),and continu-ously cultivated(5±34years)cropping sites on two soil types(Eutric Gleysol,Kairanga silt clay loam and Gleyic Luvisol,Marton silt loam).These sites,in the Manawatu region of North Island New Zealand,were selected to provide a range in duration of cultivation. Soil samples were obtained from the seven sites (Table1)located16±40km north west of Palmerston56S.Saggar et al./Soil&Tillage Research58(2001)55±68North,New Zealand(40821H S,175839H E).The Kair-anga soils are developed from quartzo-feldspathic alluvium with a predominant mica±vermiculite mine-ralogy,and the Marton soils formed from loess domi-nated by halloysite,vermiculite and inter-layered hydrous micas.Their proper location and soil classi-®cation are given in Table1.The sites have a tempe-rate humid climate with mean annual air temperatures of12.1and12.98C,and mean annual rainfall of864 and943mm,respectively.The average rainfall dis-tribution at Kairanga and Marton sites is high(270and 330mm)in winter(June±August)(Southern Hemi-sphere)and low in spring,summer and autumn sea-sons(230and200mm).The mean monthly air temperatures ranges between15and208C during summer and between8.4and10.28C during winter. The sites were farmers'®elds and had50±60years of pastoral(ryegrass/clover)land-use before being brought under cultivation.All cropping sites were conventionally cultivated up to250mm depth using a mould-board plough followed by levelling and roll-ing,discing and power harrowing.2.2.Soil samplingWithin each site,four random locations were cho-sen and are hereafter regarded as®eld replicates.At each location in each treatment(Table1),25cores (25mm diameter,0±10and10±20cm depths)were taken at approximately even spacings in an area of ca. 50m2in September1997,before spring cultivation and sowing.The cores were pooled,®eld-moist soils (À10kPa)were sieved(<2mm)soon after collection, and sieved soils were stored at48C.These`fresh'®eld-moist,sieved samples were used for soil micro-bial biomass C and N analysis and the incubation study.For soil microfaunal studies®ve®eld replicates, each comprising®ve cores(ca.25mm diameter,0±10cm soil depth)were also collected at the same time from each site.Soil bulk density was determined from undisturbed(100mm)soil core samples at0±100and 100±200mm depths and particle size distribution by pipette method.boratory incubationField-moist subsamples(equivalent to25g oven-dry soil)of each treatment replicate were placed in beakers and then incubated in1.8l glass jars contain-ing vials with10ml CO2-free water,avoiding any loss of water during incubation.The jars were tightly sealed.Respiration was measured as CO2production at weekly or fortnightly intervals for112days.On each measurement occasion,a1ml headspace sample was collected through a septum in the lid from each jar,and the CO2concentration was measured by gasTable1Land-use,soil classi®cation,location and particle size distribution for the selected sitesSoil Land-use Symbol Soil classification Grid reference(NZMS260)aNZ USDA FAOKairanga silty clay loam b Permanent pasture KPP c Typic Orthic Gley Typic Endoaquept Eutric Gleysol S2******* 5years maize K5C d Typic Orthic Gley Typic Endoaquept Eutric Gleysol S2******* 16years maize K16C Typic Orthic Gley Typic Endoaquept Eutric Gleysol S2******* 34years cropping K34C Typic Orthic Gley Typic Endoaquept Eutric Gleysol S2*******Marton silt loam Permanent pasture MPP Argillic Perched-gley Pallic Typic Endoaqualf Gleyic Luvisol R23113205 7years cropping M7C Argillic Perched-gley Pallic Typic Endoaqualf Gleyic Luvisol R2309517920years cropping M20C Argillic Perched-gley Pallic Typic Endoaqualf Gleyic Luvisol R23112201a Speci®es the farm easting and westing location on New Zealand Map Series(NZMS).b Particle size distribution(g kgÀ1);Kairanga:sand90±120,silt510±530,clay400±420,and Marton:sand80±100,silt650±660,clay 240±260.c Site renewed8years ago(1989)following wheat(Triticum sp.);before this under long-term ryegrass(L.perenne L.)and clover (Trifolium sp.).d Sites K5C and K16C were under continuous maize(Zea mays L.);M7C had5year maize 2year barley(Hordeum vulgare L.);M20C had2/3maize and1/3barley with winter oats(Avena sativa L.)or ryegrass.S.Saggar et al./Soil&Tillage Research58(2001)55±6857chromatography(GC8700,Thermal Conductivity Detector,Carle Instruments,USA).The jars were ¯ushed with ambient air and resealed for the next measurement.A set of controls(jars without soil)was used as a background reference.2.4.Soil chemical analysesAir-dried samples were used for the total C and N analyses.Total C in soils was analysed by a combustion method(Induction Furnace,Leco,St. Joseph,MI).Total soil N was determined by semi-micro Kjeldahl digestion followed by NH4 ÀN mea-surements in the digests by an autoanalyser procedure. Soil pH(1:2.5water)and oxalate extractable Fe,Al, Si were determined according to Blakemore et al. (1987).2.5.Soil biochemical measurements2.5.1.Microbial biomass C and NSoil microbial biomass C was determined by a fumigation±extraction method.Fumigated and non-fumigated soils were extracted with0.5M K2SO4for 30min(1:5soil:extractant ratio),and the extracts analysed for C by TOC5000(Shimadzu,Kyoto) analyser.The C obtained from the fumigated samples minus that from the non-fumigated samples was taken to represent the microbial-C¯ush and converted to microbial biomass C using the relationship:microbial C 10X41C flush.For soil microbial biomass N deter-minations K2SO4extracts from fumigated and non-fumigated samples were digested in0.165M K2S2O8 for30min at1218C,and NO3À-N and NH4 -N were measured by autoanalyser.Microbial N was estimated by the relationship:microbial N 10X45N flush.2.5.2.N mineralisationMineral-N(NO3À-N NH4 -N)was determined in the sieved®eld-moist soil in2M KCl(1:10,w/v) before and after the incubation by N mineralised was the difference between112-day incu-bated and0-day mineral-N values of each soil.2.5.3.Metabolic quotientThe metabolic quotient(q CO2)or speci®c re-spiration rate,was calculated as the ratio between the CO2-C produced per hour averaged over the second and third week incubation period and the microbial biomass C measured at the beginning of incubation.2.6.Soil microfaunaThe soil samples were extracted using Whitehead and Hemming trays,and total counts of enchytraeids and nematodes were made under a stereo-microscope, before®xation by addition of boiling8%formalde-hyde(Yeates and Bongers,1999).Nematodes from each replicate were identi®ed to genus and population estimates converted to a per square metre basis; nematodes were grouped into feeding types(Yeates et al.,1998).An average of103specimens per sample was identi®ed.The following indices of the nematode fauna were calculated,their rationale being given in Yeates and Bongers(1999):(1)proportion of the nematode fauna in each of the six feeding groups;(2)ratio of bacterial-feeding nematodes to total microbial-feeding nematodes(i.e.,bacterial-feeding nematodes fungal-feeding nematodes) B a B F ;(3)Shannon±Weiner diversity H H Àp i log e p i , where p i is the proportion of individuals in the i th taxon,and B and F are the populations of bacterial-and fungal-feeding nematodes.2.7.Data analysisUnless otherwise stated,soil chemical and bio-chemical results were expressed on the basis of the oven-dry(1058C)weight of the material.The experiments were pseudo-replicated with respect to treatments(Table1)only.Means of four replicates in each®eld n 4 and standard errors of the means were calculated for organic C,total N and total P,microbial biomass C,N and P and metabolic quotient.Decomposition data were subjected to an analysis of variance to determine the statistical sig-ni®cance of treatment effects,using SYSTAT7.0for Windows software package and the general linear model procedure.The signi®cance of differences for decomposition with time among treatments was estimated using repeated measures ANOV A.Calcu-lated microfaunal populations and indices were ana-lysed using ANOV A,and Fisher's LSDs calculated. The5%probability level is regarded as statistically signi®cant.58S.Saggar et al./Soil&Tillage Research58(2001)55±683.Results and discussion3.1.Changes in soil organic C and NPasture soils had more total organic C,total N and mineral N than did the cultivated soils(Table2). Cultivation of pastures for up to34years resulted in a30±60%decline in soil organic C and N.The rate of decline varied with the length of cultivation and soil type.The C:N ratios within a soil type were wider under cultivation than under pasture(Table3),indi-cating slightly greater decline in N than C upon cultivation.Most soil C and N was lost during the initial5±7year(short-term)cultivation;subsequent loss was slow.This pattern of decline in organic C and N is consistent with the results from several other studies(Carter,1991;Sparling et al.,1992;Shepherd et al.,2001).The concentration of organic matter in a given soil depends on the balance between C input and decomposition rates.The immediate loss of organic matter at conversion of pasture to cropping is partly attributed to more exposed surfaces to aeration by cultivation and to increased access of labile residues to soil microorganisms.Cultivation also favours decom-position of SOM through the breakup of soil aggre-gates with the exposure of previously inaccessible organic matter to microbial attack(Haynes,1999; Shepherd et al.,2001).Further,the annual input of C to the soil is higher under pasture than under grain cropping,Saggar et al.(1997)having shown below-ground annual C inputs under pasture to vary between 2.5and5.5Mg haÀ1depending upon soil fertility,and 1.2and2.9Mg haÀ1under cereals(Whipps,1990).3.2.Changes in soil biochemical propertiesThe MBC and MBN contents ranged from261to 1585mg C kgÀ1and41to251mg N kgÀ1,respec-tively,in the Kairanga soil,and from397to 1157mg C kgÀ1and65to283mg N kgÀ1,respec-tively,in the Marton soil(Table2).The concentrations of MBC and MBN were considerably greater in pasture soils than the cultivated soils.In both soils, the MBC and MBN showed a consistent decrease with continuous cropping(Table2).However,the differ-ences in MBC between pasture and cultivated soils were slightly more pronounced than organic C.While the decline in organic C from cultivation was up to 60%,MBC declined up to83%.This resulted in signi®cant changes in microbial quotient(MBC as a proportion of organic C)(Table3).The pasture soils had the highest microbial quotient(3.17and2.70%) that declined with cultivation.The long-term(34 years)cultivated soil had the lowest microbial quotient (1.29%).The remaining short-term(5and7years)and medium-term(16and20years)cultivated soils had intermediate values(1.44±2.22%).Both these mea-sures of C and N availability to micro¯ora appear to separate the soils on the basis of cultivation period. Our results support the®ndings of Carter(1986) that changes in microbial biomass contents are indi-cative of changes on soil organic C,and MBC is a sensitive indicator.The microbial quotient`nor-malises'biomass data from soils with different con-tents of organic C.Generally,if a soil is being exploited,the microbial quotient will decrease, whereas the reverse is true in soils with enlarging pool of soil organic C.The decline in microbial quotient with cultivation,obtained in this study,sug-gested that properties associated with`soil health'in pastures are not as sustained in cultivated soils.This was expected from the greater C inputs in pasture soils than cropping soils mentioned above.The reduction in microbial quotient under cultivation is indicative of a shift in the state of equilibrium of the soil system,and is consistent with Anderson and Domsch's(1990) predictions that microbial C:organic C eventually declines in conditions where organic matter input is low,and suggests that the microbial quotient can be used as an indicator of changes in organic matter availability.Trends in metabolic quotient(q CO2)values were opposite from microbial biomass values,tending to be lower for pasture soils than for cultivated soils (Table3).Soil respiration expressed on both a soil and soil C basis was highest in the pasture soils, suggesting that a greater proportion of soil C is decomposed in these soils compared with the culti-vated soils.The differences observed in microbial biomass C in the soils under pasture and cultivation (Table2)were not paralleled by changes in soil respiration(Table3),resulting in greater microbial biomass activities under cultivated soils than under pasture soils.The q CO2evaluates the ef®ciency of soil microbial biomass in utilising the organic C compounds.S.Saggar et al./Soil&Tillage Research58(2001)55±6859Table2Some chemical and biological characteristics of Kairanga and Marton soils(0±100mm depth)under pasture and continuous cropping(meansÆS X E X;n 4)Treatment pH(1:2.5water)Bulk density(Mg mÀ3)Organic C(g kgÀ1soil)Total N(g kgÀ1soil)Biomass(mg kgÀ1soil)Oxalate extractable(mg kgÀ1soil)Mineral N(mg kgÀ1soil)C N Fe Al SiKPP a5X65Æ0X01 1.0349X9Æ0X324X7Æ0X091585Æ5251Æ127.9 4.80.641Æ3 K5C5X84Æ0X01 1.0635X0Æ0X493X1Æ0X04776Æ17143Æ7 6.4 2.30.825Æ2 K16C5X58Æ0X04 1.1333X0Æ0X492X8Æ0X05474Æ1175Æ47.2 2.5 1.123Æ2 K34C6X20Æ0X02 1.2120X3Æ0X301X8Æ0X09261Æ1541Æ47.6 1.70.414Æ2 MPP5X64Æ0X02 1.0542X8Æ0X693X7Æ0X031157Æ40283Æ68 6.3 1.90.530Æ4 M7C5X84Æ0X04 1.1424X8Æ0X612X1Æ0X06411Æ2565Æ2 4.5 1.30.415Æ2 M20C5X80Æ0X03 1.2127X2Æ0X422X2Æ0X02397Æ1965Æ9 5.2 2.00.119Æ160S. Saggar et al. / Soil & Tillage Research 58 (2001) 55±68Table3Soil C:N ratio,microbial and metabolic quotients and C and N mineralisation rates in Kairanga and Marton soils(0±100mm depth)under pasture and continuous croppingTreatment C:N ratio Microbial quotient(MBC as proportionof organic C)(%)C mineralisation rate during112day incubation Metabolic quotient(q CO2)(CO2-C respired per hourper unit biomass CÂ10À3)Mineralised N(mg kgÀ1soil) (mg(CO2-C)kgÀ1soil hÀ1)(mg(CO2-C)kgÀ1soil C hÀ1)KPP a10.6 3.170.6412.760.573121Æ21 K5C11.4 2.220.3510.000.68343Æ3 K16C11.8 1.440.339.970.89935Æ2 K34C11.5 1.290.188.700.84526Æ2 MPP11.6 2.700.7617.790.860186Æ32 M7C11.9 1.660.3212.71 1.15934Æ5 M20C12.3 1.460.3412.40 1.12442Æ4S. Saggar et al. / Soil & Tillage Research 58 (2001) 55±68 61The greater q CO2values in cultivated soils(Table3) must re¯ect an increase in the ratio of active:dormant components of the biomass.This ratio may have been modi®ed by the available substrate(more root exu-dates and®ne roots under perennial pasture compared with those under annual cropping).The low q CO2 values in soil under pasture also suggest that during mineralisation of organic matter,microbes divert less C to respiration than to new microbial biomass,caus-ing less C loss than from microbes in cultivated soils. This may indicate the presence of microbial popula-tions in pasture soils that are more ef®cient in con-serving C.While q CO2indicates microbial ef®ciency, several factors,such as qualitative changes within microbial population(e.g.,increase in the proportion of fungi),and prevalence of zymogenous over auto-chthonous microorganisms,may explain the differ-ences in q CO2between pasture and cultivated soils.A reduction in the metabolic ef®ciency(i.e.,higher q CO2)of microbial biomass resulting from conversion of pasture to continuous cultivation agreed well with previous®ndings that less diverse agroecosystems with fewer C inputs from roots and crop residues increase q CO2(Anderson and Domsch,1990).3.3.Changes in soil microfaunaOn each soil,permanent pasture had signi®cantly more total nematodes than any of the cultivation treatments(Table4).For Enchytraeidae,the depres-sion of abundance with cultivation was only signi®-cant in the Marton soil.There were no signi®cant differences in total nematodes or enchytraeids between soils or among treatments at cultivated sites (Table4).Nematode diversity,whether measured as taxa discriminated or H H,was also greatest under permanent pasture(Table5).While the overall effects were signi®cant,in Kairanga soil H H was signi®cantly lower under16years cropping than under pasture (Table5).Decreased nematode diversity is a common feature of cultivated soils(Freckman and Ettema, 1993;Yeates and Bird,1994),and increasing cultiva-tion commonly increases losses of diversity(Boag, 1988).That diversity was generally higher in Marton soil that had less clay(260g kgÀ1)than Kairanga soil (420g kgÀ1),is in keeping with previous reports(e.g., Yeates and Bird,1994).In Kairanga soil,bacterial-feeding nematodes were most abundant under permanent pasture and signi®-cantly less abundant after5and34years cropping (Table5).In contrast,there were no signi®cant differ-ences among the Marton sites.Both soils had signi®-cantly more fungal-feeding nematodes in the shortest cultivation treatment and showed fungal-feeding nematodes were signi®cantly depressed with longer cultivation(Table5).At all sites,bacterial-feeding nematodes were more abundant than fungal-feeding nematodes,and in each soil the greatest abundance of the combined`microbial-feeding'nematodes coin-cided with that of bacterial-feeding nematodes.The high population fungal-feeding nematodes in the Mar-ton soil cropped for7years is associated with lower organic C and total N values(Table2);these three values disrupt the`expected'trends with increasing cultivation.Bacterial-mediated decomposition path-ways are generally more rapid than fungal-mediated pathways.In both our soils,bacterial feeders make their greatest relative contribution at the sites with the longest history of cultivation and,although overall ANOV A gives highly signi®cant effects,only one treatment in each soil differs signi®cantly from pas-ture(Table5).The sites with the longest history of cultivation also had the lowest microbial biomass C contents(Table2),the lowest values of microbial biomass C as a proportion of organic C and,generally, the highest values of q CO2(Table3).Whereas Marton soil had most predacious nema-todes under permanent pasture(Table5),in Kairanga soil they were most abundant in the34-year cultivated site.While the difference in response between soilsTable4Soil microfaunal populations in Kairanga and Marton soils underpasture and continuous cropping(mÀ2in0±100mm soil depth)aSite Total nematodesÂ103EnchytraeidaeKPP b28166843K5C18583079K16C18612395K34C15162737MPP274813002M7C17491368M20C19731334LSD p 0X05 3755459p(ANOV A)<0.0010.007a For each soil the maximum values are in italic.b See Table1.62S.Saggar et al./Soil&Tillage Research58(2001)55±68con®rms the®ndings of Yeates et al.(1998),the underlying mechanism is not yet known.Further, the signi®cant increase in predacious nematodes at the34-year site correlates with a marked decline in the standing crop of both bacterial-feeding nematodes (Table5),and biomass C and N(Table2).This suggests top-down pressures may be controlling these populations.Permanent pasture had signi®cantly more plant-feeding nematodes than did cropped sites on both soils;there were no signi®cant differences among the cropping treatments(Table5).Helicotylenchus dominated in the pasture on Kairanga soil,while Meloidogyne juveniles and Pratylenchus were the most abundant genera in the pasture on Marton soil. Plant-associated nematodes,which are assumed to feed on epidermal cells and root hairs,were signi®-cantly more abundant in cropping treatments than in pastures and had their greatest abundance in the Marton soil cropped for20-year(Table5).Across a series of soils,Yeates(1999)found the proportion of bacterial-feeding nematodes as a proportion of bacte-rial feeders fungal feeders plant-associated nema-todes increased with increasing human intervention.In the present soils the ratio was greatest under pasture (0.863and0.631in Kairanga and Marton soils, respectively)and decreased with cultivation,with the lowest values corresponding to the shortest periods of cultivation.While different mechanisms may be operating in these soils compared with the nine soils considered by Yeates(1999),it is also possible that there may also be signi®cant time-of-year effects. Relatively large dorylaimoid nematodes comprise the omnivorous feeding group(Yeates et al.,1998), and in both soils their abundance was greatest under permanent pasture(Table5).This result is in agree-ment with that of Freckman and Ettema(1993)who found omnivorous nematodes comprised1.8%of the nematode fauna under conventional tillage,2.03±2.99%under various cropping regimes and4.28±4.33%under alfalfa(Medicago sativa)and other no-till regimes.3.4.Soil C and N mineralisationThe CO2-C respired from the Kairanga and Marton (0±10cm)soils during the112-day incubation was 1.79and2.14g kgÀ1for the pasture soils,and0.50±0.98and0.89±0.95g kgÀ1,for soils under cultivation, respectively(Fig.1).Soil C mineralisation rates (under controlled conditions)were twice as much (ca.15mg(CO2-C)kgÀ1per day soil)in the pasture as in the5±20year cultivated soils(ca.7mg(CO2-C)kgÀ1per day soil),and were further reduced to one-half(ca.3.5mg(CO2-C)kgÀ1per day soil)in the34-year cultivated soil.During112days,the soils lostTable5Diversity and abundance of nematode feeding groups in Kairanga and Marton soils under pasture and continuous cropping(Â103mÀ2in 0±100mm soil depth)aSite H H b Taxa Bacterialfeeding FungalfeedingMicrobialfeeding cBacterial/microbial dPredacious PlantfeedingPlantassociatedOmnivorousKPP e2.0914.814518315350.931185415147536 K5C 2.0311.296414311070.861141 3.6276331 K16C 1.439.812787713560.94650.622.0249184 K34C 1.9511.0566805661.0004770.0204269 MPP2.2015.0629807090.888310611289830 M7C 1.9510.85512037540.71937.514.5373570 M20C 1.9512.2857719280.92428.082.0682252L S Dp 0X05 0.26 1.94375803750.087132.9126.4137256p(ANOV A)0.0090.0020.0070.330.0070.003<0.001<0.001<0.0010.011a For each soil the maximum values are in Italic.b Shannon±Weiner diversity index(see Yeates and Bongers,1999).c Microbial feeding bacterial feeding fungal feeding.d Bacterial feeding/microbial feeding.e See Table1.S.Saggar et al./Soil&Tillage Research58(2001)55±6863。
防治土壤流失英文作文

防治土壤流失英文作文英文:Soil erosion is a major problem that affectsagricultural productivity and the environment. It is caused by factors such as wind, water, and human activities suchas deforestation and overgrazing. To prevent soil erosion, there are several measures that can be taken.One effective method is to plant vegetation on the soil. This can be done by planting trees, shrubs, or grasses. The roots of these plants help to hold the soil in place and prevent it from being washed away by rain or blown away by wind. In addition, the vegetation helps to absorb water and prevent runoff, which can cause erosion.Another method is to use terracing. Terracing involves creating steps on a slope and planting vegetation on each step. This helps to slow down the flow of water and prevent it from washing away the soil. Terracing is particularlyeffective on steep slopes where erosion is more likely to occur.Conservation tillage is also an effective method for preventing soil erosion. This involves leaving crop residue on the soil after harvest rather than plowing it under. The residue helps to protect the soil from erosion by acting as a barrier to wind and water. In addition, conservationtillage helps to improve soil health by increasing organic matter and reducing soil compaction.In conclusion, soil erosion is a serious problem that can be prevented through various measures such as planting vegetation, terracing, and conservation tillage. By taking action to prevent soil erosion, we can protect our agricultural productivity and the environment.中文:土壤流失是影响农业生产和环境的主要问题。
- 1、下载文档前请自行甄别文档内容的完整性,平台不提供额外的编辑、内容补充、找答案等附加服务。
- 2、"仅部分预览"的文档,不可在线预览部分如存在完整性等问题,可反馈申请退款(可完整预览的文档不适用该条件!)。
- 3、如文档侵犯您的权益,请联系客服反馈,我们会尽快为您处理(人工客服工作时间:9:00-18:30)。
Agricultural Sciences in China2009, 8(6): 709-716June 2009Effects of Soil Water Content on Cotton Root Growth and Distribution Under Mulched Drip IrrigationHU Xiao-tang, CHEN Hu, WANG Jing, MENG Xiao-bin and CHEN Fu-hongAgricultural College, Shihezi University, Shihezi 832003, P.R.ChinaAbstractThe relation between soil water content and the growth of cotton root was studied for the scheme of field water and cotton yield under mulched drip irrigation. Based on the field experiments, three treatments of soil water content were conducted with 90%θf , 75%θf , and 60%θ f (θf is field water capacity). Cotton roots and root-shoot ratio were studied with digging method, and the soil moisture was observed with TDR (time domain reflector), and cotton yield was measured. The results indicated that the growth of cotton root accorded with Logistic growth curve in the three treatments, the cotton root grew quickly and its weight was very high under 75%θ f because of the suitable soil water condition, while grew slowly and its weight was lower under 90%θf due to water moisture beyond the suitable condition, and the root weight was in between under 60%θf . For the three water treatments, the cotton root weight decreased with soil depth, and decreased more significantly in deeper soil layer with the soil moisture increasing. And the ratio of cotton root weight in 0-30 cm soil layer to the total root weight was the highest under 75%θ f. The cotton root system was distributed mainly in the soil of narrow row and wide row mulched with plastic film, and little in the soil outside plastic film. The weight of cotton root was the highest in the soil of narrow row or wide row mulched with plastic film under 75% θ f. Root-shoot ratio decreased with the soil moisture increasing. The soil water content affected cotton yields, and cotton yield was the highest under 75%θf . The higher soil moisture level is unfavorable to the growth of cotton root system and yield of cotton under mulched drip irrigation. Key words: mulched drip irrigation, cotton (Gossypium hirsutum L.), soil water content, rootINTRODUCTIONSoil moisture status affects the growth, sha pe, structure, physiological function, and water uptake characteristics of crop root system as well as rootshoot ratio (Zhang et al. 1997; Li et al. 2000; Yang et al. 2007). It indicated that certain soil moisture would positively affect the increasing of root system (Turner and Begg 1981), and the position of lateral root depended on the water content of different soil layers (Taylor and Klepper 1974; Hu et al . 2006).This paper is translated from its Chinese version in Scientia Agricultura Sinica.With water stress, the root activity of crop would be feeble (Zhang Y Q 2006), and would further affect distribution of root system, plant growth, and crop yield. The root system distributed in deep soil layer was beneficial for the plant to avoid premature decrepitude and to the yield increase (Wang et al. 1997). To make effective strategy for the scheme of field water, it is necessary to study the relationship between the status and distribution of soil moisture and the increasing of crop root system. As a local irrigation technique, mulched drip irrigation is characterized by limited soil wetting pattern and higher irriga-Correspondence HU Xiao-tang, MSc, Associate Professor, E-mail: hooxiaotown-203@© 2009, CAAS. All rights reserved. Published by Elsevier Ltd. doi:10.1016/S1671-2927(08)60269-271 0HU Xiao-tang et al.tion frequency. In addition, the covered plastic film results in elevation of soil temperature, which leads to effective movement of soil water (Zhang Y P 2006). With this unique irrigation technique and soil wetting pa ttern, the cotton root system grows strongly. Previous studies (Wei et al. 2002; Hu and Li 2003; Fang et al. 2007; Cai et al. 2002) revealed that the cotton root system under mulched drip irrigation, distributed mainly in the shallow soil layer i n t he fi l m c ove r e d l a nd, a nd i nc r e a s e d i n hydrotropism. These studies contribute greatly to the evaluation of the effectiveness of soil water. The mulched drip irrigation technique for cotton had been developed in China for less than 10 years. That is why most researches in this area focused on the vertical distribution of root system and cotton yield; while very few reported on the horizontal distribution and growth of root system and shoot development. Therefore, it is necessary to carry out more studies in this area. The current study focuses on the vertical and horizontal distribution of root system of cotton and its shoot development as well as the yield under mulched drip irrigation. The samples used were treated in 3 different soil moisture levels. The purpose is to obtain scientific data for the scheme of field water and cotton yield under mulched drip irrigation.Test designCotton crop (Xinluzao 13) was planted on April 23, 2004, and April 22, 2005, field was fertilized with nitrogenous fertiliz er (urea, 589.6 kg ha -1 ) a nd diammonium orthophosphate (421.1 kg ha-1) during the cotton growing period. The transparent film with width of 120 cm covered 4 rows (N-S direction), the cotton crops were planted in row spacing 30 cm + 60 cm + 30 cm, with plant spacing 10 cm. The planting adopted the method of sowing at low soil moisture and watering for seedling. Cotton was watered with mulched drip irrigation technique. Two drip lines were laid in narrow rows under each film sheet (i.e., one tube controls 2 rows of cotton crop, Fig.1). The single-factor random experiment design included three treatments at soil moisture 90%θf, 75% θf, and 60% θf, where θf is field water capacity. Each experimental plot was segregated by a plot of 170 cm in width, which was planted with identical cotton variety. The treatments of soil moisture were carried out from the full-bud stage with four replications per treatment. The irrigation water and interval were decided according to change of soil water content, and calculated as follows: (1)MATERIALS AND METHODSTest fieldThe experiments were carried out in the Experimental Station (longitude 86°03´E, latitude 45°19´N) of College of Agriculture, Shihezi University, Xinjiang, China, in 2004-2005. This area has an annual rainfall 199 mm, annual evaporation 1 537.5 mm, annual temperature 6.6°C, and underground water table beneath land surface of 10 m. The experimental plot was 0.059 ha in 2004 and 0.049 ha in 2005. The soil was loam texture with content of physical clay (< 0.01 mm) 43%, drought bulk density 1.54 g cm-3, porosity 40.5%, and filed water capacity 30% (volumetric water content). The soil was pH 7.85 and contained 1.07 g kg-1 total nitrogen, 16.7 mg kg-1 available phosphorous, 194 mg kg-1 available potassium, and 3.48 g kg-1 total salt.Where, m denotes the irrigating water quota (mm); p, the percentage of wetted soil (60% in this case);θf, the field moisture capacity (30% in this case);θi, the soil moisture at certain depth (%); Δhi, the depth of soil sampled (m). To ensure soil moisture was kept in the designed level under weather conditions of the present year, the irrigation interval for 90%θf, 75%θf , and 60%θf treat-Fig. 1 Layout of mulched drip irrigation for cotton.© 2009, CAAS. All rights reserved. Published by Elsevier Ltd.Effects of Soil Water Content on Cotton Root Growth and Distribution Under Mulched Drip Irrigation71 1ments was 2 days, 4-6 days, and 6-10 day, respectively. The irrigating water quota were determined by equation (1).Observation items and methodA time domain reflector (TDR) was employed to measure the soil water content in the soil narrow row and wide row mulched with plastic film. The measurement was conducted at each 10 cm of soil layer from land surface to the 60 cm soil depth once every 5-6 days, and further measurements were conducted before and after irrigation. A digging method was employed to measure the root system. In 2004, the measurement was conducted at the full-bloom, full-boll, and boll-opening stages, while in 2005, the measurement was conducted at the seedling, budding, full-bloom, full-boll, and boll-opening stages. For each treatment group, 4 stocks (2 from an inside row and 2 from an outside row) of cotton crop that grew well with full seedlings and could represent the others, were selected and the roots were dug out. The width of the excavated hole was ranged from the center of the soil outside plastic film to the center of the wide row mulched with plastic film. The length of the hole was 2 times the stock space, and the depth was 80 cm. The slicing was made at every 10 cm depth.led to higher water content in the soil layer of 50-60 cm in narrow row mulched with plastic film. In the 90% θf treatment, the water content of soil in narrow row and wide row mulched with plastic film is similar and constant. In the case of 75%θf treatment (Fig.2-C and Fig.2-D), the water content of narrow-row soil mulched with plastic film fluctuates between 18% and 30%, and is higher than that of wide-row soil mulched with plastic film which is 15-22%. Since the water content of all soil layers is lower than field water capacity, there is no water percolation into deeper layer. As for 60% θf treatment (Fig.2-E, F), the water content of 0-60 cm soil layer in narrow-row and widerow soil mulched with plastic film is 8-20% and 1522%, respectively, and shows relatively large fluctuation, which indicates water supply through irrigation can not meet water consumption by crops. The vertical distribution of water content of soil for different treatments varies greatly. It seems that 75%θf treatment results in the most ideal distribution of water. Under 60%θf treatment, the water content of deep soil layer is relatively low, while 90%θf treatment results in high water content of surface soil layer.Effect of soil moisture on cotton root growthAs shown in Fig.3, root biomass accumulates in the S-shaped curve. Soil moisture is largely related to the growth of root system. At 45-70 days after seedling, dry matter of root system increases very fast, at rates of 0.1121, 0.1293, and 0.1044 g/stock/ day for 90% θ f, 75% θf, and 60% θf treatment groups, respectively. After this period (70 days after seedling), the growth rates are 0.0073, 0.0520, and 0.0323 g/stock/day for 90% θ f, 75% θf, and 60% θf treatment groups, respectively. This indicates that the cotton root grows quickly, and the root weight is the highest under the soil water treatment of 75%θ f because of the suitable soil water condition. The 90%θf treatment, however, results in an excessively moisturized soil containing more water but less air, which hinders the growth of root system, because cotton crops will avoid absorbing water (Yan 2007). As for the 60% θ f treatment, the data are between those of the other treatments. The growth of root system in all treatment groups shows little difference before the full-RESULTSSpatial distribution of soil water content under different treatmentsThe water content of naked soil outside plastic film is very low in all treatments. However, the water content of soil in narrow row mulched with plastic film is different from that in wide row mulched with plastic film. As shown in Fig.2-A and Fig.2-B, under 90% θ f treatment, the water content of surface soil layer (0-10 cm) of narrow row mulched with plastic film is larger than field water capacity (30%) due to frequent irrigation and condensation of water vapor beneath film. In addition, since irrigated water is more than that consumed by cotton, the water accumulates in the soil layer of 50-60 cm, and even percolates into deeper soil, this© 2009, CAAS. All rights reserved. Published by Elsevier Ltd.71 2HU Xiao-tang et al.Fig. 2 Soil water content with plastic film covering (2005).bloom stage, but varies significantly at the boll-opening stage, which matches with the typical logistic equation (Table 1).Effect of soil moisture on cotton root vertical distributionAn apparent “ T” distribution can be seen in the vertical distribution of root system. The weight of roots in the 0-30 cm soil layer takes a large part of the total weight of the entire root system in the three treatment groups. Among them, the proportion of roots in the total rootFig. 3 Cotton root growth (2005).© 2009, CAAS. All rights reserved. Published by Elsevier Ltd.Effects of Soil Water Content on Cotton Root Growth and Distribution Under Mulched Drip Irrigation71 3weight in this soil layer is 83.16-90.45% in the 75%θf treatment group, and 75.22-78.83% and 82.04-86.23% in the 60%θf and 90%θf treatment groups, respectively. Obviously, the ratio of cotton root weight in 0-30 cm soil layer to the total root weight was the highest under 75%θf treatment. In the soil layers deeper than 30 cm, the cotton root weight reduces significantly with soil depth. The root weight in different soil layers can be fitted using the y = A × exp(- Bx ) curve (Miao et al . 1998; Plaut et al . 1996; Hu et al . 2002), which results in the following regression equation (Table 2), where “y”, “x”, “A” and “B” stand for root weight (g m-3), depth (cm), constant, and declining rate, respectively. By comparing the mathematical models for root weight distribution at different stages of growth, it is seen that, during the same period of growth, different water treatments produce different B values (declining rate) in the root distribution model. A smaller B value means the less significant tendency that root weights would decrease with increase of depth, or a relatively large proportion in terms of the weight of deeper roots taking up in the total weight of the entire root system. The results of our study show that the B value of the 90% θf treatment group is the largest, followed by that of the 75%θf and 60%θf treatment groups. This means that 60%θf treatment results in insufficient soil moisture,Table 1 Cotton root growth model (2005)Treatment 90%θ f 75%θ f 60%θ f Root growth model y = 4.6039/[1 + 529.5210exp(-0.1195x )] y = 9.0271/[1 + 28.2297exp(-0.0416x )] y = 6.4469/[1 + 28.3459exp(-0.0482x )] Coefficient r = 0.9990** r = 0.9794** r = 0.9802**and consequently, the root has to dive deeper into the soil for water. On the other hand, 90%θ f treatment results in excessive moisture in the 50-60 cm soil layer (Fig.2). In this case, the root does not need to dive into deeper soil layer because of avoiding absorbing water. As a result, the root shows a shallow vertical distribution.Effect of soil moisture on cotton root horizontal distributionAn apparent hydrotropism can be seen in the horizontal distribution of root system of cotton plants, largely due to the belt-shaped distribution of the field soil moisture under the mulched drip irrigation. As shown in Fig.4, from the full-bloom stage to the boll-opening stage, the root systems of all the 3 treatment groups mainly distribute in the narrow and wide rows mulched with plastic film, with the root weight being 34.56-52.13% of the gross weight, but the parts distributed in the soil outside plastic film take up only 3.31-15.50% of the gross weight. This result matches with the moisture distribution shown in Fig.2. In the 60% θf treatment, the root weight of narrow-row plants is significantly higher than that of wide-row plants. In the 90% θf treatment group, the root weight of both narrow and wide row plants shows little difference. As for the 75% θf treatment group, the root weight is larger than those of the other 2 treatment groups in the soil narrow row or wide row mulched with plastic film, with the difference being small at the boll-setting stage; but large at the bollopening stage. In addition, from the bloom stage to the boll-opening stage, there is a large difference in the increase of root weight in the soil of narrow-row, wide-x is days after cotton emergence, y is root weight per plant (g), flower-boll opening stage r0.01, 6 = 0.834. **Extremely significant difference at P 0.01. The same as in Table 2.Table 2 Cotton root distribution model at different growth stagesGrowth stage Full-bloom stage Treatment 90%θ f 75%θ f 60%θ f 90%θ f 75%θ f 60%θ f 90%θ f 75%θ f 60%θ f Root distribution model y = 1917.02exp(-0.1025x ) y = 2053.29exp(-0.1020x ) y = 1006.34exp(-0.0691x ) y = 3902.99exp(-0.1534x ) y = 3310.98exp(-0.1322x ) y = 3004.27exp(-0.1415x ) y = 2618.40exp(-0.1183x ) y = 3620.68exp(-0.0938x ) y = 1230.58exp(-0.0512x ) Coefficient r = 0.9944** r = 0.9926** r = 0.9805** r = 0.9908** r = 0.9970** r = 0.9830** r = 0.9902** r = 0.9972** r = 0.9811**Boll stageBoll opening stageFlower-boll opening stages r0.01, 6 = 0.834.© 2009, CAAS. All rights reserved. Published by Elsevier Ltd.71 4HU Xiao-tang et al.row mulched with plastic film, and soil outside plastic film among different treatment groups (Table 3), with the 90% θf treatment group showing the least difference and 75% θf treatment group showing the largest increase of root weight in the narrow-row soil mulched with plastic film.Effect of soil moisture on cotton root-shoot ratioSoil moisture can affect the growth, distribution, water uptake characteristics of root system, and the growth of crop shoot. Different soil moisture can lead to different proportions of shoot to root in cotton crops. The result of our study indicates that cotton crops of the same treatment group demonstrate different root-shoot ratio in different stages of growth (Table 4). From the seedling stage to the boll-opening stage, the root-shoot ratio reduces gradually. In the boll-opening stage, the ratio becomes higher to a cer-tain degree, largely due to the premature decrepitude of shoot and falling of leaves. And the ratio shows a tendency of decreasing with the increase of soil moisture. Cotton crops of 90% θ f treatment group have the lowest root-shoot ratio. In the full-bloom stage, cotton crops of 60% θ f treatment group have the largest root-shoot ratio. After the full-bloom stage, cotton roots of 75% θ f treatment group grow faster and have the largest root-shoot ratio than that of other treatment groups.Effect of soil moisture on cotton yieldTable 5 shows the effect of soil moisture on cotton crop yield. The soil in 90% θf treatment keeps excessive moisture, so that the 0-60 cm soil layer is under bad aeration, and 0-30 cm soil layer under low temperature, which is not suitable for the growth of root system, impairs cotton shoot growth and yieldFull-bloom stageBoll stageBoll opening stageFig. 4 Horizontal distribution of root weight of cotton at different growth stages (2005). Table 3 Cotton root weight difference in flower-boll opening stagesYear 2004 Treatment 90%θ f 75%θf 60%θf 90%θf 75%θf 60%θf Increase of cotton root weight (%) Wide row 10.36 ± 1.84 44.58 ± 2.75 38.29 ± 1.46 2.89 ± 0.45 46.74 ± 3.01 41.18 ± 7.89 Narrow row 30.52 ± 4.11 53.11 ± 5.23 42.38 ± 2.60 21.41 ± 1.24 49.31 ± 3.37 40.52 ± 3.97 Land 2.97 ± 0.26 21.69 ± 4.51 3.81 ± 0.67 -0.71 ± 0.22 34.81 ± 8.96 5.28 ± 1.562005increase. The yield of 60%θf treatment group is also low. Although this treatment can ensure good aeration in the 0-60 cm soil layer and relatively high temperature in the 0-30 cm soil layer, insufficient water supply impedes the growth of the shoot and yield of cotton. Cotton crops in the 75% θ f treatment group have the highest yield because this treatment offers the mostTable 4 The root-shoot ratio of cotton under different treatmentsTreatment 90%θ f 75%θ f 60%θ f Seedling stage 0.32 ± 0.04 0.32 ± 0.04 0.32 ± 0.04 Budding stage 0.22 ± 0.02 0.22 ± 0.02 0.22 ± 0.02 Full-bloom stage 0.13 ± 0.01 0.17 ± 0.02 0.19 ± 0.02 Boll stage 0.08 ± 0.01 0.10 ± 0.02 0.09 ± 0.01 Boll opening stage 0.09 ± 0.01 0.13 ± 0.01 0.12 ± 0.02The root-shoot ratio of cotton was measured in seedling, budding, full-bloom, boll and boll opening stages, corresponding to 17, 45, 70, 92 and 149 days after cotton emergence.© 2009, CAAS. All rights reserved. Published by Elsevier Ltd.Effects of Soil Water Content on Cotton Root Growth and Distribution Under Mulched Drip Irrigation71 5Table 5 Yield and yield component of cotton under different treatments (2005)Year 2004 Treatment 90%θ f 75%θf 60%θf 90%θf 75%θf 60%θf Boll numbers 6.58 ± 0.61 a 6.70 ± 0.54 a 5.65 ± 0.38 b 5.40 ± 0.97 b 6.69 ± 1.32 a 5.88 ± 0.93 ab Boll weight (g) 5.22 ± 0.15 b 5.46 ± 0.30 a 5.44 ± 0.47 a 4.97 ± 0.39 b 5.18 ± 0.55 a 5.02 ± 0.47 ab Lint percentage (%) 39.92 ± 1.20 40.41 ± 0.73 42.40 ± 0.81 39.13 ± 2.69 41.56 ± 0.48 41.51 ± 0.69 a a b a a a Seed cotton yield (kg ha-1 ) 4 794 ± 210 a 4 922 ± 182 a 4 460 ± 171 b 4 647 ± 381 b 5 273 ± 240 a 4 758 ± 289 b2005The small letters in the same column express significant difference at 0.05 level.suitable moisture, temperature, and aeration that are necessary for the growth of both root system and shoot.DISCUSSIONThe 90% θf treatment leads to excessive moisture and bad aeration of soil. According to a previous study (Tian et al. 1999), a flooding environment causes reduction of cytokinin in root cells and slow growth of root system. In our study, cotton root of the 90%θ f treatment group revealed the growth feature of escaping from moisture surroundings and had rather shallow distribution of root system. Plants of the 60%θf treatment group demonstrate premature decrepitude at the late development stage and higher root-shoot ratio. The 75%θf treatment can ensure a suitable environment for the growth of root system and prevent excessive growth of the stems and leaves. This treatment is also economic and practical. It was reported that drought has larger impact on the plant shoot than flooding, and flooding had larger impact on the plant root system (Dong et al. 2003). However, this selective impact was not seen in our study. Our results indicate that cotton crops of the 90% θf and 60% θf treatment groups have basically the same effect on net weight of root and shoot, as compared to 75% θf treatment group. Soil temperature is an important factor for the growth of root system of cotton crop (Burke and Upchurch 1995; McMichael et al. 1996). Covered by film, soil has relatively high temperature, which promotes early development of root system. The root system has higher water uptake capability, but distributes rather shallow and mainly in the surface soil, with larger proportion of root weight in the narrow row. According to Weng et al. (1983), the distribution of root system in soil can be enlarged by increasing soil temperature. In our study, 90% θf treatment group caused dense shoot,relatively low soil temperature, and smaller horizontal distribution of root weight; while 60%θf treatment resulted in sparse shoot, relatively high soil temperature, and higher horizontal distribution of root weight than 90% θf treatment group. However, in this treatment, due to water stress, the horizontal distribution of root weight was less than that of 75%θf treatment group.CONCLUSIONSoil moisture has significant impact on the growing speed of cotton root system. The cotton root grew quickly and the root weight was very large under 75%θf treatment because of the suitable soil water condition; the root grew slowly and the root weight was the lowest under 90%θf treatment because the moisture supply was beyond the suitable soil water condition; and the root weight was in medium under 60%θf treatment. A T-shaped distribution is seen in the vertical distribution of root system for all treatment groups, and the cotton root weight decreased more significantly in deeper soil layer with the soil moisture increasing. Compared with other treatment, the ratio of cotton root weight in 0-30 cm soil layer to the total root weight was the highest under 75%θf treatment, and the lowest under 60%θf. The cotton root system was distributed mainly in the soil mulched with plastic film, and little in the soil outside plastic film, which offers a proof for hydrotropism of root system. Compared with other treatment, the cotton root weight was the highest in the soil mulched with plastic film under 75%θf treatment. From the fullbloom stage to the boll-opening stage, 90%θf treatment group caused little increase of root weight, while 75%θf treatment group brought the largest increase of root weight in the soil of narrow row mulched with plastic film. The root-shoot ratio of cotton was decreased with the soil moisture increasing. Soil water content af-© 2009, CAAS. All rights reserved. Published by Elsevier Ltd.71 6HU Xiao-tang et al.fected cotton yields, and the cotton yield was the highest under the soil water treatment of 75%θf.Experiment Botany, 36 , 303-312. Miao G Y, Yin J, Zhang Y T, Zhang A L. 1998. Study on root growth of main crops in North China. Acta Agronomica Sinica, 24 , 1-6. (in Chinese) Taylor H M, Klepper B. 1974. Water relations of cotton. I. Root growth and water use as related to top growth and soil water content. Agronomic Journal, 66, 584-588. Tian X L, Yang P Z, He Z P, Li P M. 1999. Changes of endogenous hormones in root exudates and leaf of cotton and the relation between root and growing stem. Journal of China Agricultural University, 4, 92-97. (in Chinese) Turner N C. Begg J E. 1981. Plant-water relations and adaptation to stress. Plant Soil, 58 , 97-113. Wang F H, Wang X Q, Liu S Y, Wang X L. 1997. A view on relation between distribution of crop root and yield. Journal of Shandong Agricultural Science, (4), 48-51. (in Chinese) Wei C Z, Ma F Y, Lei Y W, Li J H, Ye J, Zhang F S. 2002. Study on cotton root development and spatial distribution under film mulch and drip irrigation. Acta Gossypii Sinica, 14 , 209214. (in Chinese) Weng H Y, Yin J S, Chen Q E, Ren P H. 1983. Effects of soil temperature on cotton root and shoot growth. China Cotton , 10 , 37-38. (in Chinese) Yan X L. 2007. Principle and Practice on Plant Roots Biology. Science Press, Beijing. pp. 28-29. (in Chinese) Yang S Y, Yan P, Mei X Y, Sun X B. 2007. Effects of different soil water deficits on roots of winter wheat. Journal of Triticeae Crops, 27 , 309-313. (in Chinese) Zhang A L, Miao G Y, Wang J P. 1997. Relations of plant root to soil water. Crop Research , 2 , 4-6. (in Chinese) Zhang Y Q. 2006. Researches on Roots Growth and Regulation of Several Grain Crops. China Agricultural Scientech Press, Beijing. pp. 22-27. (in Chinese) Zhang Y P. 2006. Thermodynamics of Soil Water. Science Press, Beijing. pp. 46-64. (in Chinese) Plaut Z, Carmi A, Grava A. 1996. Cotton root and shoot responses to subsurface drip irrigation and partial wetting of the upper soil profile. Irrigation Science, 16 , 107-113. (Edited by ZHAO Qi)AcknowledgementsThis work was financially supported by the National Natural Science Foundation of China (50569004).ReferencesBurke J J, Upchurch D R. 1995. Cotton rooting patterns in relation to soil temperatures and thermal kinetic windows. Agronomy Journal, 87 , 1210-1216. Cai H J, Shao G C, Zhang Z H. 2002. Water demand and irrigation scheduling of drip irrigation for cotton under plastic mulch. Journal of Hydraulic Engineering, 11 , 119-123. (in Chinese) Dong H Z, Li W J, Tang W, Li Z H, Zhang D M. 2003. Effects of water-deficit and water-logging on some physiological characteristics of cotton seedlings. Acta Botanica Borealioccidentalia Sinica, 23 , 1695-1699. (in Chinese) Fang Y X, Zhao C Y, Chuan Z Q, Sheng Y, Lin Q H. 2007. Root distribution characteristics of cotton in different drip irrigation amounts irrigation under mulched. Journal of Soil and Water Conservation, 21 , 96-100. (in Chinese) Hu S L, Zheng D M, Deng C G, Wan S M. 2006. Study on root system development ability of cotton with different cultivation way. Research of Soil and Water Conservation , 13 , 115-116. (in Chinese) Hu X T, Li M S. 2003. Effect of trickle irrigation under sub-film on the soil conditions of rhizosphere in cotton. Chinese Journal of Eco-Agriculture, 11 ,121-123. (in Chinese) Hu X T, Li M S, Ma F Y. 2002. Drought diagnosis indexes and drip irrigation decision making for cotton mulch. Transaction of the Chinese of Agricultural Engineering , 18 , 49-52. (in Chinese) Li S K, Wang C T, Zhang W F, Wang C Y. 2000. Effects of cultivation on cotton root and shoot growth in north of Xinjiang. China Cotton, 27 , 12-13. (in Chinese) Mc Michael B L, Upchurch D R, Burke J J. 1996. Soil temperature derived prediction of root density in cotton. Environmental© 2009, CAAS. All rights reserved. Published by Elsevier Ltd.。