op100327d(ACS制药圆桌会议)
ACS患者双抗规范化治疗 ppt课件
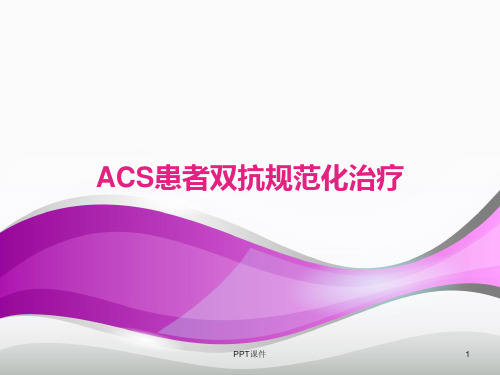
*联合终点指死亡,心梗和中风
✓ SCAAR研究,共纳入13847例直接PCI的 STEMI患者,其中9813例在到达导管室 前接受了氯吡格雷预处理.随访1年,主要 研究终点为1年死亡与MI联合终点.
✓ 两组大出血率无显著差异.
Steinhubl SR, Berger PB, Mann JT, et al. JAMA. 2002;288(19P):P2T41课1件-20.
始进入临床应用
噻氯匹定+ASA双联研究为BMS置 入术后血栓形成的预防带来新希望
PPT课件
6
急性冠脉综合征(ACS) 应尽早启动抗血小板治疗
血小板活化出现在动脉粥样硬化早期
动脉粥样硬化病变过程1
动脉粥样斑块形成期
(无临床症状,血小板已活化)
• 血管内皮损伤,血液高切变应力等因素促发血 小板活化;血小板活化启动动脉粥样硬化斑块 形成2
长期获益
8
氯吡格雷预处理,实现早期临床获益
氯吡格雷预处理降低院内和30天死亡风险
30天联合终点*1
RRR= 30%
P=0.03
院内死亡**2
RRR= 40%
P=0.048
✓ PCI-CURE研究纳入2658例行PCI的 NSTMI-ACS患者,其中1313例在院前或 在转诊医院或在转运至PCI中途接受氯 吡格雷300mg预处理.
主要心脏事件风险降低: 9.83% vs 12.35%(p<0.001)
2-8天动脉闭塞/死亡/再梗风险降低: 15.0% v急性期
(心绞痛、心梗)
• 斑块破裂,血小板粘附、活化、 聚集,血栓形成,导致ACS急性 发作2,3
Wolfgang Koenig, et al. Arterioscler Thromb Vasc Biol. 2007;27:15-26
用乙基纤维素制备酮洛芬缓释固体分散体的研究
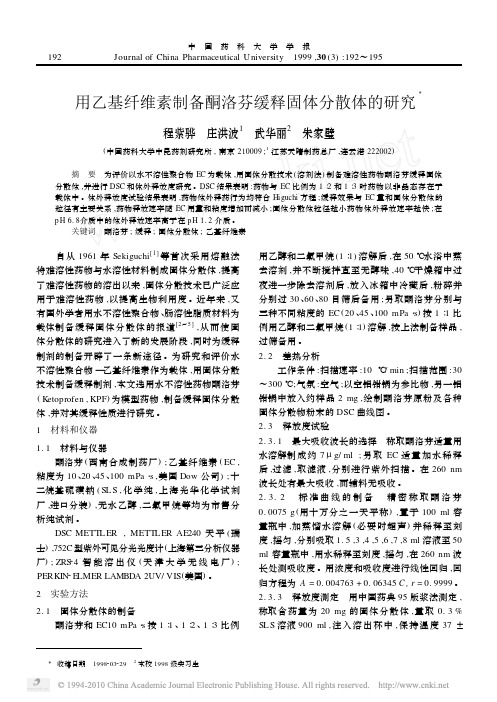
中 国 药 科 大 学 学 报Journal of China Pharmaceutical University 1999,30(3):192~195用乙基纤维素制备酮洛芬缓释固体分散体的研究Ξ程紫骅 庄洪波1 武华丽2 朱家璧(中国药科大学中昆药剂研究所,南京210009;1江苏天晴制药总厂,连云港222002)摘 要 为评价以水不溶性聚合物EC为载体,用固体分散技术(溶剂法)制备难溶性药物酮洛芬缓释固体分散体,并进行DSC和体外释放度研究。
DSC结果表明:药物与EC比例为1∶2和1∶3时药物以非晶态存在于载体中。
体外释放度试验结果表明,药物体外释药行为均符合Higuchi方程;缓释效果与EC量和固体分散体的粒径有主要关系,药物释放速率随EC用量和粘度增加而减小;固体分散体粒径越小药物体外释放速率越快;在p H6.8介质中的体外释放速率高于在p H1.2介质。
关键词 酮洛芬;缓释;固体分散体;乙基纤维素 自从1961年Sekiguchi[1]等首次采用熔融法将难溶性药物与水溶性材料制成固体分散体,提高了难溶性药物的溶出以来,固体分散技术已广泛应用于难溶性药物,以提高生物利用度。
近年来,又有国外学者用水不溶性聚合物、肠溶性脂质材料为载体制备缓释固体分散体的报道[2~5],从而使固体分散体的研究进入了新的发展阶段,同时为缓释制剂的制备开辟了一条新途径。
为研究和评价水不溶性聚合物—乙基纤维素作为载体,用固体分散技术制备缓释制剂,本文选用水不溶性药物酮洛芬(K etoprofen,KPF)为模型药物,制备缓释固体分散体,并对其缓释性质进行研究。
1 材料和仪器1.1 材料与仪器酮洛芬(西南合成制药厂);乙基纤维素(EC,粘度为10、20、45、100mPa・s,美国Dow公司);十二烷基硫磺钠(SL S,化学纯,上海光华化学试剂厂,进口分装),无水乙醇,二氯甲烷等均为市售分析纯试剂。
DSC METT LER,METT LER AE240天平(瑞士),752C型紫外可见分光光度计(上海第三分析仪器厂);ZRS24智能溶出仪(天津大学无线电厂); PERKIN2E LMER LAMBDA2UV/VIS(美国)。
HPLC—ELSD法测定心脉通软胶囊中三七皂苷R1和人参皂苷Rg1,Rb1的含量
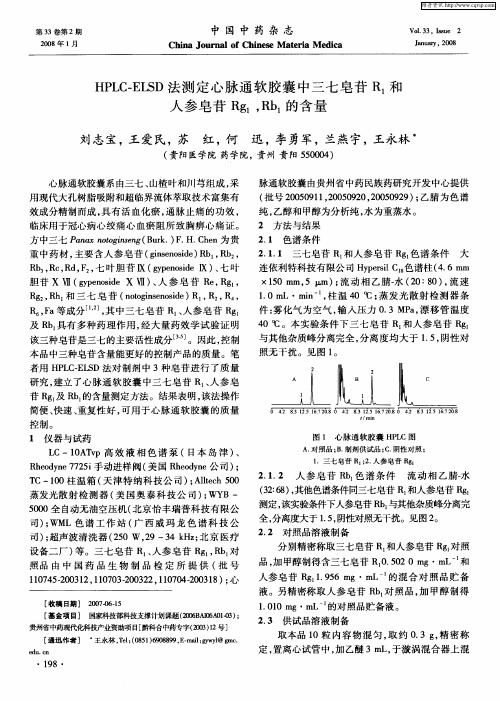
H L — L D法测 定 心 脉通 软 胶囊 中三七 皂苷 R 和 P CE S 人参 皂苷 R , b 的含量 g R
刘志 宝, 王爱 民,苏 红 , 何 迅 , 勇军,兰燕宇 , 李 王永林
( 阳医学 院 药学 院 , 州 贵 阳 500 ) 贵 贵 504 心 脉通软 胶囊 系 由三七 、 山楂 叶和川芎 组成 , 采 脉 通软 胶囊 由贵州 省 中药 民族药研 究 开发 中心提供 ( 号 20 0 1 ,059 020 02 ) 乙腈 为 色谱 批 0 59 12002 ,0 599 ; 纯, 乙醇和 甲醇为 分析 纯 , 为重蒸 水 。 水
维普资讯
第3 3卷第 2期
20 0 8年 1月
中 国 中 药 杂 志
Chn o r a fCh n s ae i e ia i a J u n lo ie eM tra M d c
Vo . 3,Is e 2 13 su
J n ay 0 8 a u r ,2 0
L C—lA v O Tp高 效 液 相 色 谱 泵 (E本 岛 津 ) t 、
R ed n 7 5 手 动进样 阀 ( 国 R ed n 公 司 ) h oy e7 2 i 美 h oy e ; T C一10柱 温 箱 ( 0 天津 特 纳 科 技 公 司 ) Ah c 0 ; leh 50
2 方法 与结 果
用现代大孔树脂吸附和超临界流体萃取技术富集有 效 成分精 制而 成 , 具有 活 血 化瘀 , 脉止 痛 的功 效 , 通
临床用 于冠心 病心 绞 痛 心 血瘀 阻 所 致胸 痹 心 痛 证 。 方 中三 七 P nxn t is g B r、 F H. h n为 贵 aa oo ne ( uk ) . C e g n 重 中药 材 , 要 含人 参 皂 苷 ( isns e R 。 R 主 g e oi ) b , b , n d
氯吡格雷CURRENT研究
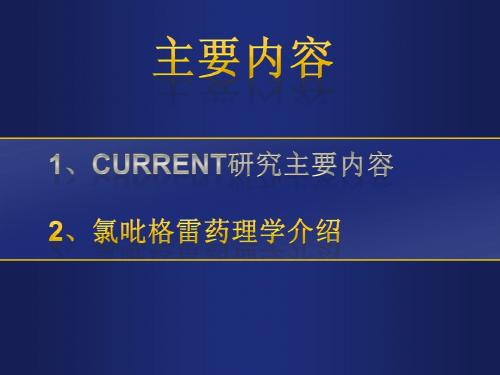
ACS抗血小板带来的获益在接受PCI治疗的 患者中更为显著
相对危险降低(RRR)
PCI CURE: 波立维 300/75 mg v 安慰剂 (CVD/MI) 30%1 非PCI 19%2
STEMI: 波立维300/75 mg v 安慰剂 (CVD/MI)
TRITON: 普拉格雷 v 波立维 300/75mg (CVD/MI/卒中)
1. Mehta SR et al. Am Heart J 2008;156:1080–1088e1.
波立维剂量组1
治疗组
第 1天 (负荷量)
剂量*
第 2–7天 (维持量) 第 8– 30天 (维持量)
高剂量组
8片75mg片剂 (600mg)
4片75mg片剂 (300mg) 和4片安慰剂
2片 75mg 片剂 (150mg)
首次症状发生后24小时内随机化入组 (2 X 2 析因): 波立维:剂量加倍 (600 mg,继以150 mg/d x 7d ,随后 75 mg/d) vs 标准剂量 (300 mg 继以75 mg/d) ASA: 高剂量 (300-325 mg/d) vs 低剂量 (75-100 mg/d)
背景
波立维
• • • •
波立维300 mg 继以75 mg /d降低所有ACS和PCI患者 的主要心血管事件 现有的数据提示波立维负荷剂量和维持剂量加倍可产 生更高和更快的抗血小板作用
阿司匹林 欧洲和北美的 ASA 使用剂量存在差异
目前尚无大规模RCTs比较ASA高剂量 (300-325 mg) 与低剂量 (75-100)分别在接受PCI治疗的ACS患者中 疗效
46%3
19%5
9%4
未评估
1. Mehta SR, et al. Lancet 2001; 358(9281):527-33. 2. Fox KAA, et al. Circulation 2004;110:1202-8 3. Sabatine MS, et al. JAMA 2005; 294(10):1224-32. 4. Chen ZM Lancet 2005;366:1607-21 4. Boersma E et al. Lancet 2002; 359:189 5. Wiviott S et al. N Engl J Med 2007; 357: 2001–15.
一种布洛芬中警示结构物质的液相色谱检测方法[发明专利]
![一种布洛芬中警示结构物质的液相色谱检测方法[发明专利]](https://img.taocdn.com/s3/m/506d83c6af45b307e87197e8.png)
专利名称:一种布洛芬中警示结构物质的液相色谱检测方法专利类型:发明专利
发明人:丰宇胜,郑忠辉,吴辉,王志萍,雷天莉
申请号:CN201910438502.5
申请日:20190524
公开号:CN110068633A
公开日:
20190730
专利内容由知识产权出版社提供
摘要:本发明提供了一种测定布洛芬中三个警示结构物质的液相色谱检测方法,所述的杂质分别为氯酮、缩酮、重排酯(布洛芬生产工艺中带氯中间体)。
本法采用高效液相色谱法,色谱柱为十八烷基硅烷键合硅胶柱,柱温为36~44℃;检测波长为氯酮260±5nm/缩酮、重排酯220±5nm;流速为1.8~2.2ml/min;流动相为有机相乙腈占总体积比例为65~75%,水相占总体积比例为25~35%,磷酸加入量占总体积比例为0.04~0.06%;各杂质的专属性、线性及范围、精密度、耐用性等试验均良好;本方法设计合理,流动相配制简单,运行时间短,节约成本,检测方法科学、客观,能简便快捷地检测布洛芬中警示结构物质,可用于布洛芬样品的质量控制,具有实用价值。
申请人:山东新华制药股份有限公司
地址:255086 山东省淄博市高新区技术产业开发区化工区
国籍:CN
代理机构:青岛发思特专利商标代理有限公司
代理人:耿霞
更多信息请下载全文后查看。
ESC冠心病DAPT指南

抗血小板治疗是把双刃剑,改善缺血的同时出血风险也会增加。 缺血和出血风险平衡一直是心血管临床医生关注的焦点, 它不仅是整个双联抗血小板治疗过程中的重点, 也是心血管医生在实际临床操作中需要熟悉和掌握的难点。
1、DAPT获益与风险评估
• 由于持续抗血小板治疗与增加的出血风险相关,因此有必要将这 一风险(增加出血)与潜在获益(降低支架血栓、降低自发心梗) 进行选快速起效的新型P2Y12抑制剂, • 将PCI前P2Y12抑制剂 “预治疗”提高为I类推荐。
新型P2Y12抑制剂的禁忌证
• *替格瑞洛禁忌证: 颅内出血病史或正在发生的出血。
• **普拉格雷禁忌证: 颅内出血史,缺血性卒中或短暂性缺血发生史或正在发生出血; 普拉格雷不推荐用于年龄≥75岁或体重<60 kg的患者。
2017ESC冠心病DAPT指南
• 2017年8月26日, • 欧洲心脏病学会(ESC)首次更新了《ESC冠心病患者双抗治疗指南》, • 指南是由欧洲心脏病学会与欧洲心胸外科学会(EACTS)共同编写。
• 2017年 • 是双联抗血小板治
疗(DAPT)用于 PCI患者的第21年。
• 基于35个临床随机 研究、
03 P2Y12受体抑制剂的换药问题
3. P2Y12受体抑制剂的换药问题
• 一直以来,阿司匹林联合氯吡格雷双抗治疗在临床上应用广泛。 • 自 2007 年 TRION 研究和 2009 年 PLATO 研究结果后, • 替格瑞洛和普拉格雷两种新型 P2Y12 受体抑制剂被应用于临床
并引起广泛的探讨和争议.
• ACS患者制定DAPT治疗类型和时长主要原则: • 考虑选择何种P2Y12受体拮抗剂、 • 何种预处理方案、 • PCI术后治疗时长 • 患者术后管理。
赛诺菲产品综合题库

赛诺菲产品综合题库波立维1,CURE研究是一个什么样的研究?氯吡格雷和美托洛尔用于心肌梗死的试验2,关于COMMIT研究结果描述正确的是:氯吡格雷75mg/日联合ASA标准治疗显著降低中国NSTEMI患者28天死亡风险氯吡格雷75mg/天显著降低中国STEMI药物治疗患者28天缺血性事件相对危险氯吡格雷75mg/日增加随访至28天时致命或非致命性出血风险。
每治疗1百万住院患者2-3周,氯吡格雷就可以挽救4000个生命,并防止另外5000次严重血管事件3,CAPRIE研究亚组分析显示,针对既往缺血性卒中和心梗史的患者,波立维较阿司匹林进一步降低心梗、缺血性卒中和血管性死亡相对危险度达:14.9%31%8.7%20%4,《中国缺血性脑卒中和TIA二级预防指南2022》指出“从二级预防的角度看,对脑卒中患者进行科学的危险分层尤为重要,比如,采用()脑卒中危险评分。
”ABCD2TIMI25%,15%5根据CAPRIE研究显示,波立维75mg/天较阿司匹林降低()胃肠道出血风险,及()胃肠道不适。
25%,16%26%,15%26%,16%6()研究证实对于实施PCI手术的患者,与12个月相比,药物洗脱支架患者使用波立维24个月更为显著地预防支架迟发血栓形成,提高了患者生存率。
CURRENTCREDOTYCOONPCI-CURE 7针对致残性卒中患者(NIHSS>3),应当如何应用波立维进行抗血小板治疗?阿司匹林长期单抗使用波立维+阿司匹林长期双抗波立维单抗长期使用波立维75mg和阿司匹林双抗21天+波立维单抗至3个月8UA/NSTEMI患者如住院期间植入药物支架者,除使用阿司匹林外,还应使用波立维()月3691292022中国缺血性卒中二级预防指南指出,氯吡格雷可以作为()抗血小板用药;三线4四线二线10ACS发病后第()年内死亡或复发风险最高?231STICHSTICHHOPETEACHCIDE以上都是11波立维卒中定点辅导可以在一下哪个项目中进行12下列哪些活动支持波立维在卒中领域的推广TEACH院长项目13关于波立维与仿制品的区别,下列哪些是正确的?波立维属于晶型II,斜方晶型;国产仿制品属晶型I,单斜晶型;热动力学试验表明,在不同湿度、温度(包含室温)条件下,氯吡格雷晶型II具有最佳的稳定性超过10万患者临床研究及全球患者使用疗效证实:氯吡格雷75mg是动脉粥样硬化血栓疾病(ACS,IS和PAD)患者的最佳剂量选择。
最新配电设备一二次融合技术方案-版

配电设备一二次融合技术方案二零一七年五月目录1 前言 (6)1.1 总体思路和目标 (6)1.1.1 总体推进思路 (6)1.1.2 总体目标 (6)2 柱上开关一二次成套技术方案 (7)2.1 一二次成套总体要求 (7)2.2 一二次成套功能要求 (8)2.2.1 分段/联络断路器成套功能要求 (8)2.2.2 分段/联络负荷开关成套功能要求 (9)2.2.3 分界断路器成套功能要求 (11)2.2.4 分界负荷开关成套功能要求 (11)2.3 一二次成套技术要求 (12)2.3.1 总体结构要求 (12)2.3.2 分段/联络断路器成套技术要求 (13)2.3.3 分段/联络负荷开关成套技术要求 (13)2.3.4 分界断路器成套技术要求 (14)2.3.5 分界负荷开关成套技术要求 (14)2.3.6 自动化部件技术要求 (15)2.3.6.2 电压/电流互感器(传感器)技术要求 (15)2.3.6.3 控制单元技术要求 (18)2.3.7 通信及接口要求 (19)2.4 抗凝露方案 (20)2.4.1 凝露问题分析 (20)2.4.2 柱上开关抗凝露方案 (21)2.4.3 环网柜抗凝露方案 (21)2.3.4 控制电缆及插头抗凝露方案 (21)2.3.5 控制单元抗凝露方案 (22)2.5 行程开关改进方案 (22)2.5.1 产生遥信抖动的原因分析 (22)2.5.2 解决方案 (23)3 环网柜一二次成套技术方案 (23)3.1 一二次成套化方案 (23)3.2 一二次成套技术要求 (24)3.2.1 开关柜典型分类和组成 (24)3.2.2 成套设备应用技术要求 (25)3.2.2.1 成套设备整体要求 (25)3.2.2.2 抗凝露要求 (26)3.2.3 开关柜技术要求 (26)3.2.4 互感器及DTU技术要求 (28)3.2.4.1 互感器技术要求 (28)3.2.4.2 控制单元技术要求 (29)3.2.5 接口要求 (31)3.2.5.1 操作电源的配置 (31)3.2.5.2 电缆及接线端子 (31)3.2.6 通信 (32)4 环网柜一二次融合技术方案 (32)4.1 一二次融合方案 (32)4.2 一二次融合技术要求 (33)4.2.1 开关柜典型分类和组成 (33)4.2.2 一二次融合设备应用技术要求 (34)4.2.2.1 一二次融合设备整体要求 (34)4.2.2.2 抗凝露要求 (36)4.2.3 开关柜技术要求 (37)4.2.4 互感器(传感器)及DTU技术要求 (39)4.2.4.1 互感器(传感器)技术要求 (39)4.2.4.3 控制单元技术要求 (40)4.2.5 接口要求 (41)4.2.5.1 操作电源的配置 (41)4.2.5.2 电缆及接线端子 (41)4.2.6 通信 (42)4 配电线损采集模块技术要求 (42)4.1 总体要求 (42)4.1.1 用于箱式FTU的配电线损采集模块 (42)4.1.2 用于罩式FTU的配电线损采集模块 (43)4.1.3 用于一二次成套化方案DTU的配电线损采集模块 (43)4.1.4 用于一二次融合方案DTU的配电线损采集模块 (43)4.2 规格要求 (43)4.2.1 准确度等级 (43)4.2.2 参比电压 (43)4.2.3 参比电流 (44)4.2.4 标准的参比频率 (44)4.2.5 配电线损采集模块常数 (44)4.3 接口及结构要求 (44)4.3.1 脉冲输出 (44)4.3.2 RS232/RS485通信接口 (45)4.3.3 电源及功耗要求 (45)4.3.4 结构及接口定义 (46)5 投标及检测要求 (49)5.1 投标检测资质要求 (49)5.2 供应商投标资格要求 (50)5.3 供货设备与入网专业检测样机元器件一致性要求 (50)附录A 接插件电气管脚定义(柱上开关一二次成套设备) (50)附表A.1 柱上开关26芯航空插件管脚电气定义 (50)附表A.2 FTU电源/电压航空插头引脚定义 (52)附表A.3 FTU电流输入接口引脚定义 (52)附表A.4 FTU控制信号航空插头引脚定义(配弹簧机构开关) (53)附表A.5 FTU控制信号航空插头引脚定义(配永磁开关) (53)附表A.6 FTU控制信号航空插头引脚定义(配电磁机构开关VSP5) (53)附表A.7 适用于箱式FTU的配电线损采集模块接口定义 (54)附录B 接插件电气管脚定义(环网柜一二次成套设备) (55)附表B.1 DTU工作电源航空插头引脚定义 (55)附表B.2 DTU电压输入端子定义 (55)附表B.3 DTU电流输入与控制信号端子定义 (56)附表B.4 DTU配电线损采集模块接口定义 (57)附录C 接插件电气管脚定义(环网柜一二次融合设备) (58)附表C.1 间隔单元33芯矩形连接器端子和对应引线信号定义 (58)附表C.2 间隔单元脉冲输出端子定义 (61)附表C.3 间隔单元通讯输出端口定义 (62)附表C.4 间隔单元维护端口定义 (62)附表C.5 公共单元电源端口定义 (62)附表C.6 公共单元RS485接口定义 (62)附表C.7 公共单元遥信接口定义 (63)附表C.8 电源电压总线PT控制柜二次室端子定义 (63)附表C.9 电源电压总线间隔柜二次室侧端子定义 (63)附表C.10 电流传感器航插接口定义 (64)附表C.11 公共单元柜端子定义 (64)附表C.12 以太网通讯线定义 (65)1 前言1.1 总体思路和目标1.1.1 总体推进思路通过提高配电一、二次设备的标准化、集成化水平,提升配电设备运行水平、运维质量与效率,满足线损管理的技术要求,服务配电网建设改造行动计划。
一种测量切向压电常数d15的新方法
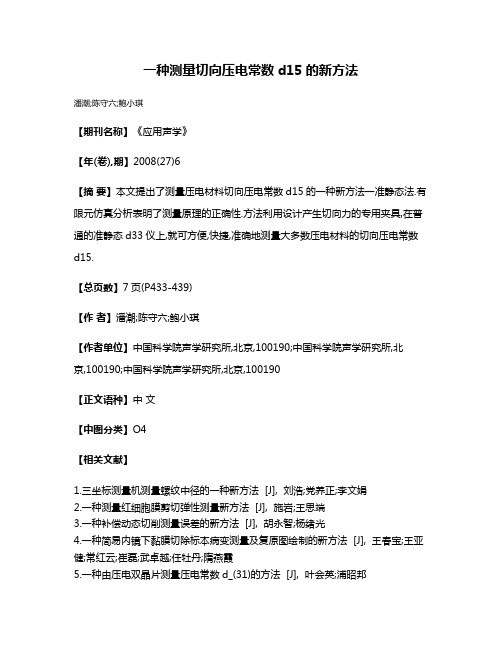
一种测量切向压电常数d15的新方法
潘潮;陈守六;鲍小琪
【期刊名称】《应用声学》
【年(卷),期】2008(27)6
【摘要】本文提出了测量压电材料切向压电常数d15的一种新方法一准静态法.有限元仿真分析表明了测量原理的正确性.方法利用设计产生切向力的专用夹具,在普通的准静态d33仪上,就可方便,快捷,准确地测量大多数压电材料的切向压电常数d15.
【总页数】7页(P433-439)
【作者】潘潮;陈守六;鲍小琪
【作者单位】中国科学院声学研究所,北京,100190;中国科学院声学研究所,北京,100190;中国科学院声学研究所,北京,100190
【正文语种】中文
【中图分类】O4
【相关文献】
1.三坐标测量机测量螺纹中径的一种新方法 [J], 刘浩;党养正;李文娟
2.一种测量红细胞膜剪切弹性测量新方法 [J], 施岩;王思端
3.一种补偿动态切削测量误差的新方法 [J], 胡永智;杨绪光
4.一种简易内镜下黏膜切除标本病变测量及复原图绘制的新方法 [J], 王春宝;王亚健;常红云;崔磊;武卓越;任牡丹;隋燕霞
5.一种由压电双晶片测量压电常数d_(31)的方法 [J], 叶会英;浦昭邦
因版权原因,仅展示原文概要,查看原文内容请购买。
施耐德电气助上海公利医院迈向“智慧”
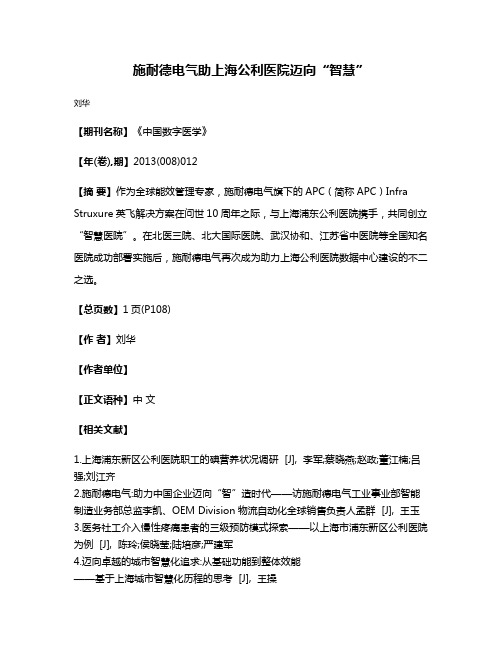
施耐德电气助上海公利医院迈向“智慧”
刘华
【期刊名称】《中国数字医学》
【年(卷),期】2013(008)012
【摘要】作为全球能效管理专家,施耐德电气旗下的APC(简称APC)Infra Struxure英飞解决方案在问世10周年之际,与上海浦东公利医院携手,共同创立“智慧医院”。
在北医三院、北大国际医院、武汉协和、江苏省中医院等全国知名医院成功部署实施后,施耐德电气再次成为助力上海公利医院数据中心建设的不二之选。
【总页数】1页(P108)
【作者】刘华
【作者单位】
【正文语种】中文
【相关文献】
1.上海浦东新区公利医院职工的碘营养状况调研 [J], 李军;蔡晓燕;赵政;董江楠;吕强;刘江齐
2.施耐德电气:助力中国企业迈向“智”造时代——访施耐德电气工业事业部智能制造业务部总监李凯、OEM Division物流自动化全球销售负责人孟群 [J], 王玉
3.医务社工介入慢性疼痛患者的三级预防模式探索——以上海市浦东新区公利医院为例 [J], 陈玲;侯晓莹;陆培彦;严建军
4.迈向卓越的城市智慧化追求:从基础功能到整体效能
——基于上海城市智慧化历程的思考 [J], 王操
5.施耐德电气助企业能耗瘦身高达30%——施耐德电气召开2010年解决方案南京站峰会 [J],
因版权原因,仅展示原文概要,查看原文内容请购买。
心房颤动和血管成形术药物获得批准上市

心房颤动和血管成形术药物获得批准上市
刘冰馨
【期刊名称】《心血管病防治知识》
【年(卷),期】2009(0)11
【摘要】@@ 美国食品药品管理局(FDA)每年会批准好几十个药物上市.其中的很多都不值得注意,例如跟现有药物差不多的仿制品、配方调整或者现有药物的复合制剂.
【总页数】2页(P38-39)
【作者】刘冰馨
【作者单位】(Missing)
【正文语种】中文
【相关文献】
1.FDA批准上市获得QIDP认证的新药概况 [J], 牛亚平;梁蓓蓓;孙湃;王睿;贾晋生
2.首个超薄药物洗脱支架Orsiro于美国批准上市 [J],
3.他汀类药物在心房颤动上游治疗中的最新认识李广平等关于他汀类药物预防房颤的研究被欧洲心脏病学大会最新心房颤动指南引用 [J], 刘彤;刘恩照
4.首个国产心脏三维标测系统获得SFDA批准上市 [J], 凌寒
5.醋酸兰瑞肽在美国获得批准上市 [J],
因版权原因,仅展示原文概要,查看原文内容请购买。
我国将对经典中成药进行疗效再评价
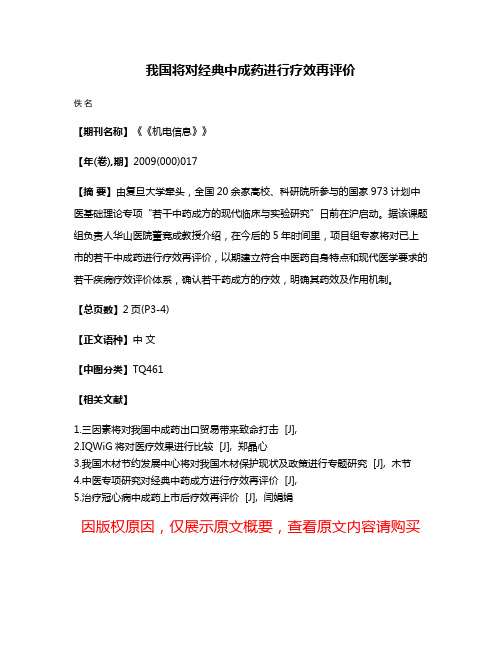
我国将对经典中成药进行疗效再评价
佚名
【期刊名称】《《机电信息》》
【年(卷),期】2009(000)017
【摘要】由复旦大学牵头,全国20余家高校、科研院所参与的国家973计划中医基础理论专项“若干中药成方的现代临床与实验研究”日前在沪启动。
据该课题组负责人华山医院董竞成教授介绍,在今后的5年时间里,项目组专家将对已上市的若干中成药进行疗效再评价,以期建立符合中医药自身特点和现代医学要求的若干疾病疗效评价体系,确认若干药成方的疗效,明确其药效及作用机制。
【总页数】2页(P3-4)
【正文语种】中文
【中图分类】TQ461
【相关文献】
1.三因素将对我国中成药出口贸易带来致命打击 [J],
2.IQWiG将对医疗效果进行比较 [J], 郑晶心
3.我国木材节约发展中心将对我国木材保护现状及政策进行专题研究 [J], 木节
4.中医专项研究对经典中药成方进行疗效再评价 [J],
5.治疗冠心病中成药上市后疗效再评价 [J], 闫娟娟
因版权原因,仅展示原文概要,查看原文内容请购买。
罗克韦尔自动化助力中国制药行业

罗克韦尔自动化助力中国制药行业
佚名
【期刊名称】《自动化博览》
【年(卷),期】2004(21)6
【摘要】11月17日,罗克韦尔自动化公司参加了第九届中国国际医药展览会暨技术交流会。
【总页数】1页(P3-3)
【关键词】中国;制药行业;罗克韦尔自动化公司;国际;技术交流会;展览会;医药
【正文语种】中文
【中图分类】TP278;F832
【相关文献】
1.自动化与制药行业的亲密接触——罗克韦尔自动化强势亮相中国国际医药展 [J],
2.自动化与制药行业的亲密接触——罗克韦尔自动化强势亮相中国国际医药展 [J], 无
3.自动化与制药行业的亲密接触——罗克韦尔自动化强势亮相中国国际医药展 [J],
4.自动化与制药行业的亲密接触——罗克韦尔自动化强势亮相中国国际医药展 [J],
5.罗克韦尔自动化:智能制造助力轮胎工业未来发展——访罗克韦尔自动化中国区
轮胎行业销售经理贾睿萌先生 [J],
因版权原因,仅展示原文概要,查看原文内容请购买。
液相色谱--荧光检测法提高黄曲霉毒素信噪比的研究
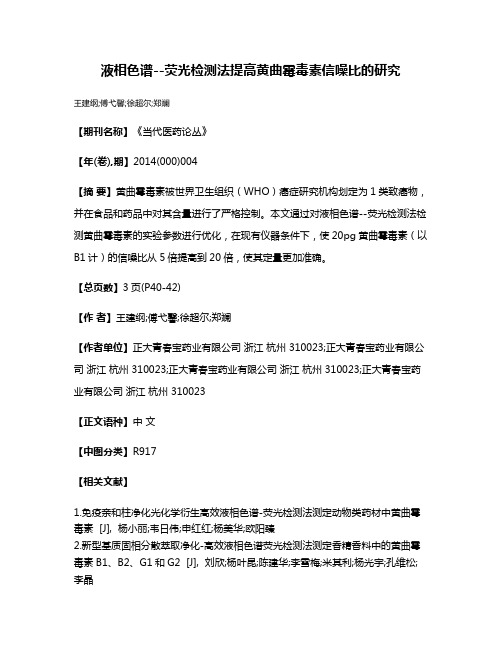
液相色谱--荧光检测法提高黄曲霉毒素信噪比的研究
王建纲;傅弋馨;徐超尔;郑斓
【期刊名称】《当代医药论丛》
【年(卷),期】2014(000)004
【摘要】黄曲霉毒素被世界卫生组织(WHO)癌症研究机构划定为1类致癌物,并在食品和药品中对其含量进行了严格控制。
本文通过对液相色谱--荧光检测法检测黄曲霉毒素的实验参数进行优化,在现有仪器条件下,使20pg黄曲霉毒素(以B1计)的信噪比从5倍提高到20倍,使其定量更加准确。
【总页数】3页(P40-42)
【作者】王建纲;傅弋馨;徐超尔;郑斓
【作者单位】正大青春宝药业有限公司浙江杭州 310023;正大青春宝药业有限公司浙江杭州 310023;正大青春宝药业有限公司浙江杭州 310023;正大青春宝药业有限公司浙江杭州 310023
【正文语种】中文
【中图分类】R917
【相关文献】
1.免疫亲和柱净化光化学衍生高效液相色谱-荧光检测法测定动物类药材中黄曲霉毒素 [J], 杨小丽;韦日伟;申红红;杨美华;欧阳臻
2.新型基质固相分散萃取净化-高效液相色谱荧光检测法测定香精香料中的黄曲霉毒素B1、B2、G1和G2 [J], 刘欣;杨叶昆;陈建华;李雪梅;米其利;杨光宇;孔维松;李晶
3.液相色谱-荧光检测法快速检测饲料中黄曲霉毒素B1 [J], 刘成模;杨曦;陈小燕;黄智成
4.食品中黄曲霉毒素B1柱后光化学衍生-高效液相色谱检测法研究 [J], 黄轩
5.固相萃取-高效液相色谱-荧光检测法测定谷物中的黄曲霉毒素B_1 [J], 张惠贤;童圆;胡西洲;彭立军;彭西甜;冯钰锜
因版权原因,仅展示原文概要,查看原文内容请购买。
复方丹参滴丸通过美国FDA的IND审评之路
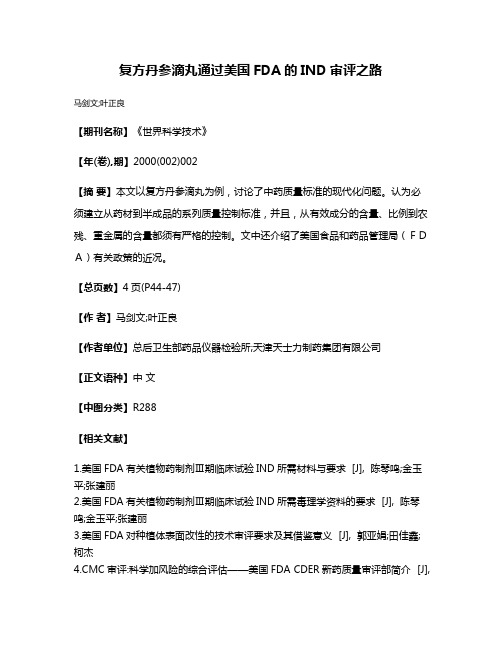
复方丹参滴丸通过美国FDA的IND审评之路
马剑文;叶正良
【期刊名称】《世界科学技术》
【年(卷),期】2000(002)002
【摘要】本文以复方丹参滴丸为例,讨论了中药质量标准的现代化问题。
认为必
须建立从药材到半成品的系列质量控制标准,并且,从有效成分的含量、比例到农残、重金属的含量都须有严格的控制。
文中还介绍了美国食品和药品管理局(FDA)有关政策的近况。
【总页数】4页(P44-47)
【作者】马剑文;叶正良
【作者单位】总后卫生部药品仪器检验所;天津天士力制药集团有限公司
【正文语种】中文
【中图分类】R288
【相关文献】
1.美国FDA有关植物药制剂Ⅲ期临床试验IND所需材料与要求 [J], 陈琴鸣;金玉平;张建丽
2.美国FDA有关植物药制剂Ⅲ期临床试验IND所需毒理学资料的要求 [J], 陈琴鸣;金玉平;张建丽
3.美国FDA对种植体表面改性的技术审评要求及其借鉴意义 [J], 郭亚娟;田佳鑫;
柯杰
4.CMC审评:科学加风险的综合评估——美国FDA CDER新药质量审评部简介 [J],
Meheb Nasr;汤玉冰
5.茚达特罗上市剂量选择的论战:美国FDA定量药理学审评的故事 [J], 尹芳;王玉珠;郑青山
因版权原因,仅展示原文概要,查看原文内容请购买。
赛多利斯在中国举办制药企业质量认证培训巡回讲座

赛多利斯在中国举办制药企业质量认证培训巡回讲座
卞斐妍
【期刊名称】《中国计量》
【年(卷),期】2009()10
【摘要】为了更好地为制药企业用户服务,更好地了解行业内的动态,2009年5月,赛多利斯在上海、天津和广州举办了制药企业质量管理培训巡回讲座。
【总页数】1页(P127-127)
【关键词】企业质量管理;制药企业;认证培训;讲座;中国;用户服务
【作者】卞斐妍
【作者单位】
【正文语种】中文
【中图分类】F273.2;F426.7
【相关文献】
1.制药企业质量认证培训会——赛多利斯为您解决认证难题 [J],
2.“制药企业质量认证培训巡回讲座”纪要 [J], 本刊通讯员
3.制药企业质量认证培训会——赛多利斯为您解决认证难题 [J],
4."制药企业质量认证培训巡回讲座"纪要 [J],
5.赛多利斯举办“制药企业质量认证培训巡回讲座” [J],
因版权原因,仅展示原文概要,查看原文内容请购买。
培哚普利对充血性心力衰竭患者校正后QT离散度的影响
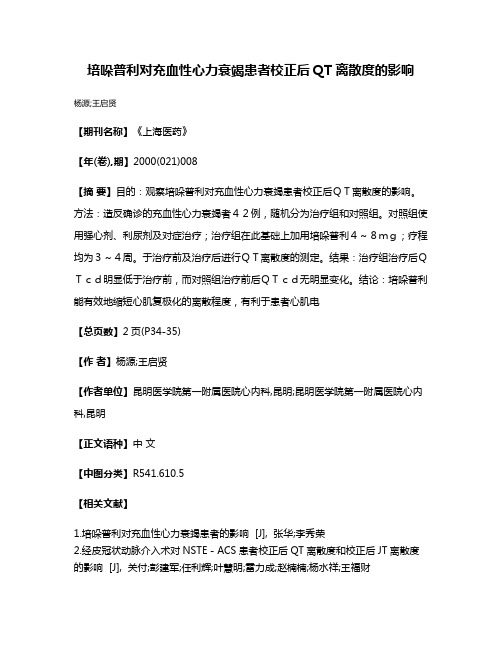
培哚普利对充血性心力衰竭患者校正后QT离散度的影响杨源;王启贤
【期刊名称】《上海医药》
【年(卷),期】2000(021)008
【摘要】目的:观察培哚普利对充血性心力衰竭患者校正后QT离散度的影响。
方法:造反确诊的充血性心力衰竭者42例,随机分为治疗组和对照组。
对照组使用强心剂、利尿剂及对症治疗;治疗组在此基础上加用培哚普利4~8mg;疗程均为3~4周。
于治疗前及治疗后进行QT离散度的测定。
结果:治疗组治疗后QTcd明显低于治疗前,而对照组治疗前后QTcd无明显变化。
结论:培哚普利能有效地缩短心肌复极化的离散程度,有利于患者心肌电
【总页数】2页(P34-35)
【作者】杨源;王启贤
【作者单位】昆明医学院第一附属医院心内科,昆明;昆明医学院第一附属医院心内科,昆明
【正文语种】中文
【中图分类】R541.610.5
【相关文献】
1.培哚普利对充血性心力衰竭患者的影响 [J], 张华;李秀荣
2.经皮冠状动脉介入术对NSTE-ACS患者校正后QT离散度和校正后JT离散度的影响 [J], 关付;彭建军;任利辉;叶慧明;雷力成;赵楠楠;杨水祥;王福财
3.培哚普利对充血性心力衰竭患者左心室重构及血流动力学的影响 [J], 赵丽霞;孟庆莲;李春祥
4.培哚普利对充血性心力衰竭患者血浆脑钠素水平的影响 [J], 周良;刘文清;朱红俊;龚少愚;苏伟
5.培哚普利联合卡维地洛对老年充血性心力衰竭患者心功能及炎性指标的影响 [J], 王美力;蔡海滨;李东升
因版权原因,仅展示原文概要,查看原文内容请购买。
- 1、下载文档前请自行甄别文档内容的完整性,平台不提供额外的编辑、内容补充、找答案等附加服务。
- 2、"仅部分预览"的文档,不可在线预览部分如存在完整性等问题,可反馈申请退款(可完整预览的文档不适用该条件!)。
- 3、如文档侵犯您的权益,请联系客服反馈,我们会尽快为您处理(人工客服工作时间:9:00-18:30)。
Key Green Engineering Research Areas for Sustainable Manufacturing:A Perspective from Pharmaceutical and Fine Chemicals ManufacturersConcepci o n Jim e nez-Gonz a lez,*,†Peter Poechlauer,‡Quirinus B.Broxterman,§Bing-Shiou Yang,^ David am Ende,||James Baird,z Carl Bertsch,0Robert E.Hannah,9Phil Dell’Orco,O Henk Noorman,b Sandy Yee,4Raf Reintjens,§Andrew Wells,2Viviane Massonneau,3and Julie Manley1†GlaxoSmithKline,Sustainability and Environment,5Moore Drive,Research Triangle Park,North Carolina,United States‡DSM Pharmaceutical Products,St.-Peter-Strasse25,4021Linz,Austria§DSM Innovative Synthesis B.V.,P.O.Box18,6160MD Geleen,The Netherlands^Boehringer Ingelheim Pharmaceuticals,Inc.,900Ridgebury Road,Ridgefield,Connecticut06877-0368,United States)Pfizer Inc.,Chemical Research and Development,Pfizer,Groton,Connecticut06340,United Statesz AstraZeneca,Essential Safety,Health and Environment,Alderley Park,Macclesfield,U.K.0Eli Lilly and Co.,Chemical Process Research and Development,Lilly Technology Center,Indianapolis,Indiana,United States9GlaxoSmithKline,Sustainability and Environment,1Franklin Plaza,Philadelphia,Pennsylvania,United StatesO GlaxoSmithKline,Research and Development,Upper Merion,Pennsylvania,United Statesb DSM Biotechnology Center PO Box425,2600AK Delft,The Netherlands4Johnson&Johnson,EHS2Compliance and Environmental Affairs,Global Pharma,200Tournament Drive,Horsham,Pennsylvania 19044,United States2AstraZeneca,Pharmaceutical Development,Bakewell Road,Loughborough,U.K.3Merck MSD Manufacturing,Z.I de Blavozy43700Saint Germain Laprade,France1ACS Green Chemistry Institute,1155Sixteenth Street,NW,Washington,DC20036,United StatesABSTRACT:In2005,the American Chemical Society(ACS)Green Chemistry Institute(GCI)and global pharmaceutical companies established the ACS GCI Pharmaceutical Roundtable to encourage the integration of green chemistry and engineering into the pharmaceutical industry.The Roundtable developed a list of key research areas in green chemistry in2007,which has served as a guide for focusing green chemistry research.Following that publication,the Roundtable companies have identified a list of the key green engineering research areas that is intended to be the required companion of thefirst list.This publication summarizes the process used to identify and agree on the top key green engineering research areas and describes these areas,highlighting their research challenges and opportunities for improvements from the perspective of the pharmaceutical industry.1.INTRODUCTIONThe pharmaceutical industry is devoted to inventing medi-cines that allow patients to live longer,healthier,and more productive lives and is committed to bringing key medicines to the patient with minimal environmental impact.The concept of Green Engineering is not new in pharmaceutical manufacturing. In recent years,the pharmaceutical industry has paid significant attention to productivity improvement,waste reduction as well as quality improvement and control in both the research and development(R&D)and manufacturing areas.This is driven not only by the consideration of cost reduction but also by the awareness of increasing sustainability of the manufacturing process.In2005,the American Chemical Society(ACS),Green Chemistry Institute(GCI),and several global pharmaceutical corporations founded the ACS GCI Pharmaceutical Roundtable (hereafter referred to as the Roundtable).The activities of the Roundtable reflect the joint belief that the pursuit of green chemistry and engineering is imperative for a sustainable busi-ness and environment.Therefore,the mission of the Roundtable is to catalyze the implementation of green chemistry and engineering into the business of drug discovery,development, and production.To achieve this mission,the Roundtable identi-fied four strategic priorities:rming and influencing the research agenda in thehigh value-added areas of green chemistry andengineering.Special Issue:Sustainable Process ChemistryReceived:December10,2010Published:February22,2011/OPRD2.Developing tools for innovation.To identify,design,and provide tools that promote innovation in green chemistry and engineering.cating leaders .To educate leaders and scientists in the merits of applying green chemistry and engineering in the pharmaceutical industry.4.Collaborating globally.To provide green chemistry and engineering expertise worldwide to pharmaceutical cor-porations and fine chemical companies.In 2007the Roundtable developed a list of key Green Chemistry research areas 1that was published as a perspective article to provide an assessment of the current state of the art in those areas,and to highlight opportunities for future improvement.As a natural follow up to the 2007work,the Roundtable has decided to develop and publish key green engineering research areas from the perspective of pharmaceutical and fine chemical manufacturers.In this paper,the process for de fining these key areas and research needs is reported,and their research chal-lenges and opportunities for improvements from the pharma-ceutical industry perspective are de fined.2.PROCESS FOR IDENTIFYING AND AGREEING ON THE KEY GREEN ENGINEERING AREASThe process started with gathering ideas from engineering,chemistry,and biotechnology representatives from the member companies collaborating in an initial brainstorming exercise.During this exercise,the ideas generated were grouped in speci fic areas that would help focus research and requirements (Figure 1).The expert group felt that all the ideas generated were high quality and worthy of research support,and that the grouped listprovided focused guidance of the industry needs while also giving additional context.The list of research areas was re fined and then prioritized by a blinded process of each member company voting for their top five areas of interest.The output of the brainstorming exercise and prioritization is shown in Table 1.One of the key messages was that there was a lot of commonality in the individual company votes.This allowed the Roundtable to quickly identify not only the 10Key Green Engineering Research Areas but also the top five priorities from the Roundtable members ’perspective.There were additional areas identi fied that did not obtain any votes during prioritization (e.g.,mass and energy integration).Once the results from the brainstorming and the initial vote were known,the member companies sought external feedback from academics and other industrial areas via presentations and discussions in conferences such as the annual meeting of the American Institute of Chemical Engineers (AIChE)2and the American Chemical Society ’s Green Chemistry and Green Engineering annual conference.3In general,the feedback re-ceived has been very positive.3.THE TOP 10GREEN ENGINEERING RESEARCH AREAS For each of the key research areas shown in Table 1,a short overview containing the description,research challenges and opportunities,its relevance,and recommendations are given.Although all of the areas are covered in this publication,it was decided that the primary focus will be on the top five research areas.3.1.Continuous Processing.Currently pharmaceutical manufacture is dominated by batch processing.Pharmaceutical manufacturing typically involves several consecutive but segmen-ted unit operations/processes which produce drug substance (orFigure 1.Process used to identify and agree on the key green engineering areas.Table 1.Key greenengineering research areas:results of the brainstorming and prioritization exercisesAPI-active pharmaceutical ingredient)and the formulated drug product,respectively.Assurance of product quality and unifor-mity is dominated by techniques involving sampling and measur-ing at discrete points(although increase in the use of inline/ real-time techniques through application of Process Analytical Technologies,PAT,has been seen in recent years).Because of the high-quality attributes of the products,manufacturing is carried out under a rigorous regulatory framework with the highest quality standards.Pharmaceutical andfine chemical manufacture can be char-acterized as an industry traditionally operating inflexible multipurpose batch plants both for API and formulated drug product.In response to a changing marketplace and business environment the pharmaceutical industry is undergoing signifi-cant changes.With increased cost-pressures,companies are looking at opportunities to lower operating costs.By some estimates,cost of goods of APIs represent on average30%of revenues,based on sampling a number of pharmaceutical manufacturers.This translates to significant potential cost sav-ings if more efficient methods can be adopted.4,5In other industries,such as confectionary or petroleum,where margins have been historically lean,continuous processing is often utilized.Continuous Processing(CP)is one aspect of process intensification in which the goal is to reduce costs,reduce the size of process equipment,improve product quality,reduce energy consumption,solvent utilization,and waste generated.A CP approach is constrained only by the limits of chemistry and physics,whereas batch processing is often constrained by equip-ment limits.When applied to pharmaceutical manufacturing and fine chemicals,the rationale for continuous processing includes several advantages:Economics.Lower cost of production can be obtained via reduced inventory,footprint,waste and emissions,and energy consumption.One example is the potentially lower cost of goods when running supercritical chemistry in supercritical solvents such as carbon dioxide and near-critical water,although uptake of this technique by pharma outside chromatography has so far been negligible.6Continuous reactors can be better suited for cryogenic reactions since the heat removal efficiency is greatly increased over a batch reactor.Quality.Operation under continuous steady state offers im-proved product quality and consistency versus batch operations which are run dynamically.This quality increase can be obtained by improved mass and heat transfer and the ability to operate more intensely at higher temperatures.In addition,process deviations can be less detrimental since there is less risk to product due to lower holdup,resulting in less product rework requirements(less raw materials,waste,and energy use).More precise control of variables such as temperature,pressure,and heat transfer can improve yields and selectivity and reduce process variance.This“steady-state”operation can be superior when compared to possible batch-to-batch variability.Also, integrating PAT can provide opportunity for real-time release of product.Finally,during development and scale up,the orders of scale up to production mode can be reduced when compared to a batch operation.One example of improved consistency and safety as compared to batch is the scale-up of a continuous enolization,oxidation,and quench process to produce 6-hydroxybuspirone.7The flow process utilized static mixers and a custom trickle bed reactor assembly to generate over100kg of API.The authors reported faster processing times as a result of higher operating temperatures,more consistent reactions via steady-state operation,and increased safety due to significantly lower inventories of flammable solvent in the presence of oxygen, when compared to the batch operation.Safety.Process safety is enhanced via smaller reactor volumes and holdup volumes of potentially hazardous reagents or sol-vents within the process.Smaller flow containment facilities(i.e., walk-in hoods)provide a reduced potential for exposure of high-potency and/or cytotoxics via processing when compared to the batch configuration.Continuous processing can enable the minimization of risks with hazardous chemistry that otherwise would have to be abandoned or heavily modified to run in batch mode.One example of safety drivers favoring continuous proces-sing is the reduced potential for explosion of flammable atmo-sphere in batch processing:Kopach et al.observed that headspace components in a batch reactor can lead to explosive conditions which is a barrier to commercial scale up.Running in a tubular reactor eliminates this headspace,allows higher tempera-tures to decrease reaction time,and lowers the amount of hazardous compounds present.8Another continuous flow appli-cation with a safety driver is continuous nitration.New nitration chemistry processes run continuously are frequently cited as offering safety benefits from significantly reduced inventories of hazardous reaction media(in the reactor volume)and thus offer significantly reduced risk of operation.9-11 Environmental.Batch processes are inherently wasteful with frequent nonvalue-added operations.One relatively recent as-pect of continuous technology is microreactor technology,which exhibits unprecedented reaction control.Microreactors combine the advantages of continuous processing with the complexity of the pharmaceuticals synthesis.12-14One example of an applica-tion is the utilization of rapid mixing and heat transfer when running a highly concentrated reaction stream,which minimizes waste.Another advantage of continuous processing is the potential for solvent reductions since the reaction can be run neat in a flow reactor or at least more concentrated.Thus, significant reduction can be realized in Process Mass Intensity (PMI).Solvent usage and emissions can also be reduced due to the lower frequency of cleaning compared to cleaning in batch operation.This would occur from the CP steady-state operations which should require occasional shutdown and cleaning.3.1.1.Research Challenges and Opportunities for Pharma-ceutical Continuous Processes.Design and development of fully integrated continuous processes requires detailed under-standing of the process so that the resultant knowledge can be used to run at steady state,providing consistent,high-quality product.Although many process unit operations are compatible with continuous mode,new approaches to chemical synthesis and reaction technology for production of APIs need to be developed,expanded,and optimized to enable continuous regime at scale.15,16For drug product production,additional research and devel-opment is required for understanding the fundamental engineer-ing of operations such as blending,granulation,and drying. Although it is assumed that the benefits of continuous operation may be more fully realized with the design of new production facilities,there is a need to develop process intensification approaches that can be applied to convert batch multipurpose facilities into continuous.There are significant challenges to continuous processing related to startups and shutdowns caused by operational issues and equipment failures(e.g.,clogging),and additional research is needed to address those issues.In general much moredevelopment work is required to extend continuous to biologics/ biopharmaceutical processing.3.2.Bioprocesses.There is increased interest in industrial biotechnology fueled in great part by global issues such as climate change,petroleum depletion,energy and food supply,biodiver-sity,etc.The applications range from high value-low volume products such as biopharmaceuticals to low value-large volume products(such as bioplastics and biofuels).Some of the enablers and drivers include the current developments in technology (molecular and synthetic biology,enzyme and cell evolution, high-throughput experimentation tools,model-driven design and development),new manufacturing concepts(integrated biorefineries,17,18),governmental mandates(biofuels)as well as opportunities from regulatory(FDA:process analytical tech-nology,quality by design)and intellectual property perspective (patent expiry resulting in novel processes for(bio)generics—also termed biosimilars).In addition,next-generation concepts, such as usage of cell-free synthesis,transgenic plants/animals as alternative production platforms may provide further improve-ments in the future.As a result,biotechnology products are strengthening their market positions,with annual sales of over$187billion.Table2 contains a short list of materials produced through bioprocesses, the volumes produced in2005-2007,and estimated market sizes.19,20Over90%of the revenues are in the(bio)pharma markets,but processing volumes are much higher in the biofuel, biochemical,food,and feed segments.Bioprocesses and biotransformations have for some time been known to contribute to highly stereo-,chemo-,and regioselective routes that can sometimes reduce the number of steps in a synthesis,lower the energy needs,and produce less green house gases(GHG)emissions21-23and hazardous waste.Numerous industrial biotransformations are in operation worldwide.24Most of these known biotransformations are used to produce inter-mediates and raw materials for the pharmaceuticalfine chemical industry.Bioprocesses are indeed a great opportunity for sustainable engineering.However,from the experiences of the authors, bioprocesses are not by definition‘greener’than the chemoca-talytic alternative.For instance,a comparison of processes using metal catalysts and one using biocatalysts for the enantioselective reduction of ketoesters in pharmaceutical synthesis was per-formed using a streamlined LCA methodology.The analysis identified some processes and reaction conditions that had the largest significance to the impacts of the synthesis.It was also concluded that whether the metal catalysts were better than biocatalysts depended mainly on the workup from the use of organic solvents and energy-intensive steps.25This example clearly expresses a need to better integrate bioprocessing design with engineering and life cycle principles to be able to develop greener,more effective and sustainable processes,which can be both chemo-or biobased,or have a hybrid structure.3.2.1.Biopharmaceuticals.A subset of bioprocesses of spe-cial interest within the pharmaceutical industry is the production of biopharmaceuticals.There are opportunities to reduce the environmental footprint and the economic cost of biopharma-ceutical processing.There have been dramatic improvements demonstrated in the performance of cell cultures,with much higher product titers and reactor productivities.26This could pave the way to much smaller and more flexible facilities with large reductions in the use of buffers,clean-in-place(CIP)and sterilize-in-place(SIP)systems.27Economic bottlenecks have shifted to downstream processing.At the same time,there are demonstrated areas for implementation of simpler and less water-and energy-intensive technologies and operations,such as membrane separations,high throughput process design,high throughput experimentation,protein crystallization,aqueous two-phase extraction,mixed-mode(resin)matrices instead of protein-A affinity chromatography,etc.As a consequence,the building of new biopharmaceutical plants(or retrofitting of existing ones)will allow a strong reduction of investment cost from the200-1000million range to50-200million range.It is envisioned that redesign of the whole biopharmaceutical manu-facturing process with much lower eco-footprint28will be feasible in the near future.The area of biopharmaceuticals has so far stayed rather remote from the recent advances in industrial biotechnologyfield.The new integrated factories(biorefineries)that convert biomass into biofuels,biomaterials,and neutraceutical/food/feed products are designed and constructed,governed by minimizingflows ofTable2.Annual production and business volumes of major bioderived products,2005-2007aproduct annual production(kton)annual market size(B$)bioethanol>50,000>10amino acids(except chemically producedD,L-methionine)>3000 3.7(41%L-glutamic acid,41%L-lysine.HCl,8%L-threonine,10%others)citric acid1,800 1.6lactic acid250>0.5ascorbic acid(vitamin C)1070.5anti-infective antibiotics>10055(160products:36%β-lactams,19%antivirals,12%quinolones,11%macrolides,22%other)industrial enzymes>100 2.3(34%detergents,27%foods,feeds16%,textiles10%,other13%)gluconic acid600.13xanthan300.4pharmacological agents<10>50(statins,cyclosporines,etc.)riboflavin(vitamin B2)50.13biopharmaceuticals<163(200products:21%EPO,11%MAbs,10%interferon,9%human insulin,50%other)a From refs19and20.raw materials,use of alternative feed-stocks based on renewable resources such as biomass,and application of clean processes with reduced solvent inventories,lower energy input,renewable catalysts,and mild conditions for reaction and separation.29 Most likely this parallel development is due to emphasis on implementing biopharmaceutical processes effectively to meet the exacting regulatory demands placed on such products.30 Hence,implementation of supply chains,rather than integration and optimization,has been the necessary focus of biopharma-ceutical process engineering.31,32However,adapting the tools of industrial biotech to biopharmaceutical processes may yield processes with lower production cost and lower environmental impact.3.2.2.Research Challenges and Opportunities for Biopro-cesses.The new‘biobased economy’will require the develop-ment of a suitable infrastructure and,like the oil-based counterpart,will demand very high efficiency,meaning that research in process engineering for the future implementation and establishment of bioprocesses is needed.In addition,research is needed that supports the timely identification of environmental,health,and safety issues to be managed within bioprocesses.This research needs to ensure that any claims of‘greenness’(i.e.,an emphasis on environmentalbenefits)are considered in the wider framework of sustainability. Attempting to assess and compare the sustainability of biopro-cesses must have a holistic scope based on life cycle thinking, which is strongly based on the output of systems engineering modeling and simulation techniques.Societal issues(food vs fuel,“land use”,GMOs)have become part of the discussion and need to be addressed in an open and constructive way.Process systems engineering(PSE)can be helpful here by providing systematic tools for quantification based on transparent assumptions and (mass/energy)balancing principles.Bioprocesses exhibit a wide applicability in the bulk,fine chemical,and pharmaceutical industries.A further integration of various communities working for the biotechnology business, with sharing of best practices and joint efforts,will undoubtedly support further growth and“greening”of the area.Opportunities in future research include:•BiocatalysisO as a replacement for inefficient or hazardous chemical reactions such as amidation and redox chemistries,which are intensively used in the pharmaceutical andfine chemical industriesO the application of biocatalysis in the production of more complex pharmaceuticals•Integration and optimization of bioprocessing design with engineering and systems thinking,especially in biopharma-ceutical(biologics)processing,so the gains achieved by the biotransformation are not lost during the workup and purification.•Integration of life cycle thinking in the design of biophar-maceutical(biologics)processes to evaluate the true (economic,environmental,and societal)sustainability and renewability of the process.•Application(extended)of process systems engineering and modeling tools to develop more rational and integrated (bio)process design,control,and improvement.33At the same time,this supports the recent FDA requirements for knowledge-based improvements and quality by design (QbD).3.3.Reactions and Separations.The manufacture of API from starting materials typically involves a multistage sequence, with each stage utilizing a series of reaction and separation steps. While the reaction step often is the‘focal point’of the stage,it frequently requires only a fraction of the time,energy,and material mass relative to the subsequent isolation.Figure2shows the frequency of common unit operations in pharmaceutical syntheses.Separations consist of approximately three-fourths of the total number of unit operations used in a process.For every 2reactions,approximately2extractions,1distillation,1crystal-lization,1product isolation,1product drying step,and0.5 nonproduct filtrations(e.g.,carbon or clarifying filtration)steps are performed.Separation steps are conducted primarily in a batch or semibatch mode,with continuous or intensified proces-sing methods seldom employed in drug manufacture.From a green engineering perspective,the separation steps across a pharmaceutical synthesis contribute a range of approxi-mately40-90%of the process mass intensity of a synthesis.In terms of energy utilization,distillation and drying steps alone often consume greater than50%of the energy requirements of a process,while frequently bottlenecking throughput.As a result, the energy and time requirements for separation steps often dwarf those required for the reaction,lead to increased facility size and energy requirements,and are the predominant con-tributor to processing energy and costs.Novel,intensified,integrated,and more energy efficient separation methods are required to drive a step change in the green and sustainable manufacture of pharmaceuticals.Instances of such technologies are often exemplified in the production of bulk chemicals.One such example is reactive distillation,35which has greatly intensified commercial processes for simple esters and ethers through integrating reaction and separation steps.How-ever,analogous methods relevant to larger-molecular-weight materials are still largely in the developmental stage.Several authors have stressed the needs for the intensification of separa-tion methods and their integration with reaction steps,35,36but few have concentrated on the specialized needs offine chemicals and pharmaceuticals,whereflexibility is critical,molecular Figure2.Frequency of unit operations used in pharmaceutical synth-eses.Derived from GlaxoSmithKline’s phase III and new product portfolio.complexity is high,and a large batch processing infrastructure is currently in place.In addition to the most typical unit operations as shown in Figure2,there are ongoing trends taking place within the pharmaceutical industry.These trends are not by any means new in other chemical industries but have been traditionally less applied in pharmaceutical separation technologies.Some of these current trends are:Process Analytical Technology(PAT).These have been ex-tensively used in pharmaceutical R&D and sometimes in the manufacturing process.Examples include the applications of FBRM/PVM(Focused Beam Reflectance Measurement/Parti-cle Vision Measurement)on crystallization monitoring and control,Raman in the determination of solute concentration, polymorph conversion and polymorph control,FTIR/NIR (Fourier Transform Infra-Red and Near Infra-Red)for solvent exchange and determination of solute concentration,mass spectroscopy to determine end-point of drying and conductivity to determine the end point of cake wash.37-39With the applica-tion of PAT tools,the data collection time is greatly shortened, and the understanding of the process is enhanced,improving the overall quality and minimizing failure.PAT can also enable the process monitoring and control for process intensification efforts.Batch to Continuous Processing.The advantages of moving from batch to continuous processing have been discussed above. In terms of separation,filtration and distillation can be easily operated in a semicontinuous mode.There are also some examples of conducting crystallization in the(semi-)continuous mode that could provide the advantages of better control of quality or particle size distribution.Cases of producing small particle size via a semicontinuous impinging jet and continuous polymorph conversion under high shear techniques have been reported.40,41Automation.Automation technologies have been extensively used in the development stages of separation processes as well as in manufacturing plants.Automation provides the opportunity for process scientists to develop and optimize with a small amount of material that generally results in a more efficient process.For instance,high throughput crystallization screening has been used in the early polymorph/salt screen to identify a suitable solid form for further development as well as to conduct polymorphism studies.42Automated solubility measurement is a common tool to screen for solvents for reaction and crystal-lization as well as for separation of impurities.Process Modeling.Mathematical modeling is a key area that chemical engineers have skills that most chemists do not share. Ideally,modeling can contribute to the greenness of the process by generating predicted process information and outcomes without physically executing large numbers of experiments; thereby minimizing waste.On the other hand,modeling can also enable efficient overall process design and contribute to the process optimization through simulation of the entire process. Examples for this include modeling/prediction for solvent selection in reaction,extraction,crystallization,and cleaning.43 Once a model is built,the effect of process operating conditions in scale-up could be predicted through simulation.3.3.1.Research Challenges and Opportunities for Reactions and Separations.Two important(but not yet greatly studied and practiced)research areas in separation technologies that can facilitate green and sustainable manufacturing are intensified separations and hybrid systems combining reaction and separation.Intensified separations will enable the transition from batch to continuous processing,while hybrid systems will help improve on selectivity.The use of multiple separation steps in countercurrent operation will lead to a significant reduction of solvent use and product loss.3.3.1.1.Development of Intensified Separations.Thefirst research challenge is the development and implementation of intensified separation technologies with highly productive modular units performing conventional tasks such as: extraction,distillation,andfiltration.This implies that a small module will be able to process a large continuousflow of material during its short residence time in that module.The modules should be small so that theyfit within an existing multipurpose plant.Although the aim is to have pharmaceutical production increasingly in continuous mode as discussed above,the phar-maceutical industry will probably continue to have campaign-based operations.Sharing the facility over several products is expected to remain beneficial,but adapting the plant to the next campaign should become much easier and faster.Mobile mod-ules allow for offline cleaning,maintenance,reconfiguration, validation,etc.The plant will have some kind of‘backbone’interface allowing these modules to‘dock’to the appropriate position in the process.Final validation of the full process should take much less time with these preprepared modules.One example of a separation used routinely in pharma that could benefit from intensification and enhancements is phase separation.Each separation step is based on the same principles:•creating a second phase•contacting both phases and allowing the component to reach a partition(thermodynamic equilibrium)over both phases•separating the two phasesDevelopments on hydrophobic/hydrophilic membrane and centrifugal separators for phase separation have the potential to result in high efficiency liquid-liquid(L/L)and gas-liquid (G/L)separation systems.However,pharma production often has to deal with liquid-solid(L/S)systems.Hence,another oppor-tunity lies in performing research that increases the availability of technologies that intensify L/S operations.For instance,the formation of suspension can be intensified and converted to continuous operation by the application of oscillatingflow systems, but this excludes the batch operation of the S/L separation and drying(API milling,formulation).3.3.1.2.Development of Hybrid Systems.Additional re-search and development on combining separation and reaction into one operation is needed.These hybrid systems should result in the in situ product removal reactor,which is a powerful tool capable of shifting chemical equilibrium or avoiding selectivity loss in case of follow-up chemistry degrading the desired product. Typical examples of such combinations are:•reactive distillation•reactive extraction•reactive crystallization(production of optically pure substances)•membrane reactorDespite many ongoing research activities in thefield,there still exist numerous technical and nontechnical barriers that hinder a wider introduction of reactive separations into industrial practice. Some research opportunities to overcome these challenges include:•research tofill specific technical gaps,such as lack of simulation and scale-up capability,lack of validated。