Signal properties of spaceborne squint-mode SAR
Unit2OutofThisWorldProject教学设计高二下学期英语牛津译林版(2020)选择

牛津译林版(2020)选择性必修第三册Unit 2 Out of This WorldProject教材分析:本节课程来自牛津译林版(2020)选择性必修第三册Unit 2 Out of This World。
本节课的主题是探索宇宙,通过学习探险家威廉·哈勃和他的伽利略太空望远镜,以及参观NASA太空探索中心,让学生了解人类对宇宙的探索和太空科学的发展。
教学目标:1. 掌握课文中的重点词汇和短语。
2. 了解威廉·哈勃的贡献以及伽利略太空望远镜的重要性。
3. 了解NASA太空探索中心的建设与发展。
4. 培养学生对宇宙科学的兴趣和探索精神。
教学重点:1. 重点词汇和短语的掌握与运用。
2. 威廉·哈勃和伽利略太空望远镜的介绍。
3. NASA太空探索中心的介绍。
教学难点:1. 威廉·哈勃和伽利略太空望远镜的相关知识的理解和运用。
2. 学生对NASA太空探索中心的建设与发展的理解和探讨。
学情分析:学生是高二年级的学生,已经具备一定的英语基础。
他们对科学和宇宙有一定的兴趣,但对相关知识了解可能较为有限。
学生可能对威廉·哈勃和伽利略太空望远镜的故事和NASA太空探索中心的发展有浓厚的兴趣,希望能通过这节课进一步了解。
教学策略:1. 引导学生观看相关视频和图片,激发学生的兴趣。
2. 组织小组讨论和合作学习,促进学生之间的互动和合作。
教学方法:1. 师生互动教学:教师以讲解和引导的方式,让学生主动参与课堂讨论。
2. 合作学习:通过小组合作和小组讨论的方式,让学生共同学习和分享。
导入环节(约5分钟):导入活动:通过展示一张风景画或者播放一个与太空相关的短视频来引起学生的注意。
导入内容:引入本节课的主题——太空。
导入活动:教师可以请学生观察画面或者视频中的元素,并提出以下问题进行导入:1. 你认为这是什么地方?2. 你对外星生物有什么了解?3. 你有过类似的太空梦吗?描述一下。
高三英语天文观测设备单选题50题
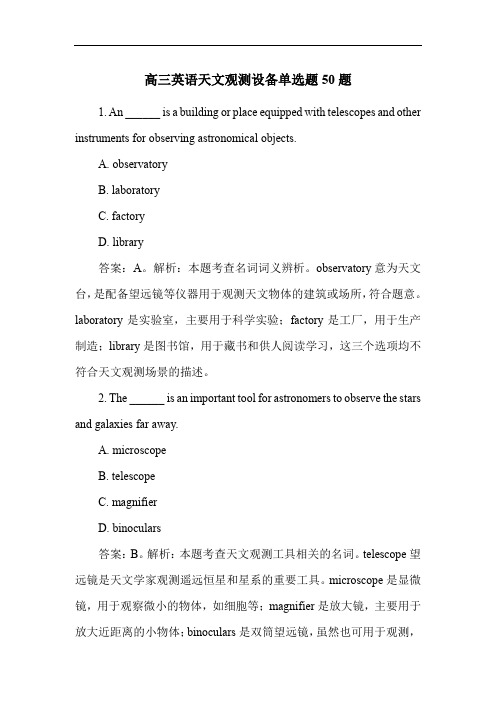
高三英语天文观测设备单选题50题1. An ______ is a building or place equipped with telescopes and other instruments for observing astronomical objects.A. observatoryB. laboratoryC. factoryD. library答案:A。
解析:本题考查名词词义辨析。
observatory意为天文台,是配备望远镜等仪器用于观测天文物体的建筑或场所,符合题意。
laboratory是实验室,主要用于科学实验;factory是工厂,用于生产制造;library是图书馆,用于藏书和供人阅读学习,这三个选项均不符合天文观测场景的描述。
2. The ______ is an important tool for astronomers to observe the stars and galaxies far away.A. microscopeB. telescopeC. magnifierD. binoculars答案:B。
解析:本题考查天文观测工具相关的名词。
telescope望远镜是天文学家观测遥远恒星和星系的重要工具。
microscope是显微镜,用于观察微小的物体,如细胞等;magnifier是放大镜,主要用于放大近距离的小物体;binoculars是双筒望远镜,虽然也可用于观测,但在天文观测中telescope更为专业和常用。
3. In the observatory, the ______ of the telescope needs to be adjusted precisely to get a clear view of the celestial bodies.A. lensB. buttonC. handleD. box答案:A。
解析:本题考查名词在天文观测设备中的部件。
The Pros and Cons of Space Exploration
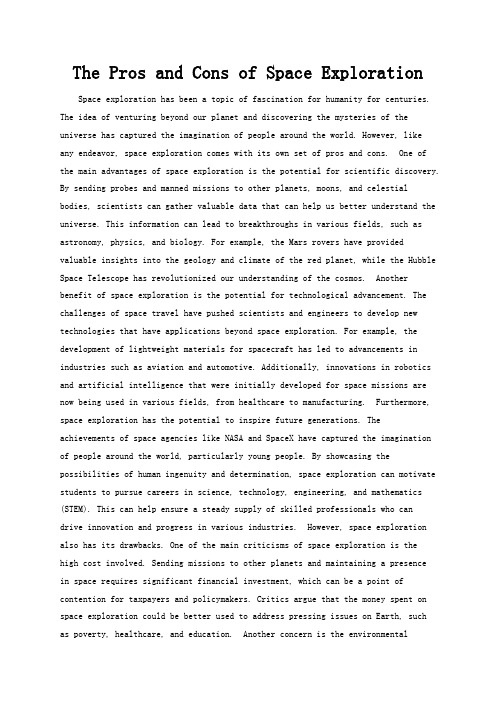
The Pros and Cons of Space Exploration Space exploration has been a topic of fascination for humanity for centuries. The idea of venturing beyond our planet and discovering the mysteries of the universe has captured the imagination of people around the world. However, likeany endeavor, space exploration comes with its own set of pros and cons. One of the main advantages of space exploration is the potential for scientific discovery. By sending probes and manned missions to other planets, moons, and celestial bodies, scientists can gather valuable data that can help us better understand the universe. This information can lead to breakthroughs in various fields, such as astronomy, physics, and biology. For example, the Mars rovers have provided valuable insights into the geology and climate of the red planet, while the Hubble Space Telescope has revolutionized our understanding of the cosmos. Anotherbenefit of space exploration is the potential for technological advancement. The challenges of space travel have pushed scientists and engineers to develop new technologies that have applications beyond space exploration. For example, the development of lightweight materials for spacecraft has led to advancements in industries such as aviation and automotive. Additionally, innovations in robotics and artificial intelligence that were initially developed for space missions are now being used in various fields, from healthcare to manufacturing. Furthermore, space exploration has the potential to inspire future generations. The achievements of space agencies like NASA and SpaceX have captured the imagination of people around the world, particularly young people. By showcasing the possibilities of human ingenuity and determination, space exploration can motivate students to pursue careers in science, technology, engineering, and mathematics (STEM). This can help ensure a steady supply of skilled professionals who candrive innovation and progress in various industries. However, space exploration also has its drawbacks. One of the main criticisms of space exploration is thehigh cost involved. Sending missions to other planets and maintaining a presencein space requires significant financial investment, which can be a point of contention for taxpayers and policymakers. Critics argue that the money spent on space exploration could be better used to address pressing issues on Earth, suchas poverty, healthcare, and education. Another concern is the environmentalimpact of space exploration. Rockets and spacecraft produce greenhouse gases and other pollutants that can contribute to climate change and harm the environment. Additionally, space debris left behind by missions can pose a hazard to satellites and spacecraft in orbit around Earth. As space exploration becomes more common, these environmental concerns will need to be addressed to ensure thesustainability of future missions. Furthermore, there are ethical considerations to take into account when it comes to space exploration. The potential for contamination of other planets and celestial bodies by Earth microbes, as well as the impact of human presence on alien ecosystems, raises questions about our responsibility as stewards of the universe. Additionally, the militarization of space and the potential for conflict over extraterrestrial resources are concerns that must be addressed to ensure the peaceful exploration of space. In conclusion, space exploration offers a range of benefits, from scientific discovery and technological advancement to inspiration for future generations. However, it also comes with challenges, such as high costs, environmental impact, and ethical considerations. As we continue to explore the cosmos, it is important to weigh these pros and cons carefully and ensure that our efforts in space benefit notonly humanity but also the universe as a whole.。
外星文明猜想(英文作文)

Certainly! Here’s an essay exploring the conjectures about extraterrestrial civilizations, delving into the scientific, philosophical, and speculative aspects of the topic. Extraterrestrial Civilizations: The Great Beyond and Our Place in the CosmosThe universe, vast and ancient, stretches its arms across 93 billion light-years of observable space, containing billions of galaxies, each with billions of stars. Within this cosmic tapestry, the question of whether we are alone has captivated human minds for centuries. This essay explores the conjectures surrounding extraterrestrial civilizations, from the scientific theories to the speculative musings that fuel our imaginations.The Drake Equation: A Mathematical Framework for SpeculationAt the heart of the search for extraterrestrial intelligence (SETI) lies the Drake equation, formulated by astronomer Frank Drake in 1961. This mathematical framework attempts to estimate the number of active, communicative civilizations in the Milky Way galaxy. Variables include the rate of star formation, the fraction of stars with planetary systems, the number of planets capable of supporting life, the fraction of those planets where life actually emerges, the fraction of those life-bearing planets that develop intelligent life, the fraction of those that develop a civilization with technology, and the length of time such civilizations release detectable signals into space. While many of these variables remain unknown, the Drake equation serves as a tool for structured speculation and highlights the immense challenge in estimating the likelihood of extraterrestrial life.The Fermi Paradox: Where Are They?The Fermi paradox, named after physicist Enrico Fermi, poses a compelling question: Given the vastness of the universe and the high probability of habitable worlds, why have we not encountered any evidence of extraterrestrial civilizations? This paradox has led to numerous hypotheses. Perhaps civilizations tend to destroy themselves before achieving interstellar communication. Or, advanced civilizations might exist but choose to avoid contact with less developed species, adhering to a cosmic form of the “prime directive” seen in science fiction. Alternatively, the distances between stars could simply be too great for practical interstellar travel or communication, making detection exceedingly difficult.The Search for TechnosignaturesIn the quest for extraterrestrial intelligence, scientists have focused on detecting technosignatures—signs of technology that might indicate the presence of a civilization elsewhere in the universe. These include radio signals, laser pulses, or the dimming of stars due to megastructures like Dyson spheres. SETI projects, such as the Allen Telescope Array and Breakthrough Listen, scan the skies for anomalous signals that could be attributed to alien technology. While no definitive technosignatures have been found to date, the search continues, driven by advances in technology and a growing understanding of the cosmos.Astrobiology: Life Beyond EarthAstrobiology, the study of the origin, evolution, distribution, and future of life in the universe, offers insights into the conditions necessary for life. Research in astrobiology has revealed that life can thrive in extreme environments on Earth, suggesting that the conditions for life might be more widespread in the universe than previously thought. The discovery of exoplanets in the habitable zones of their stars, where liquid water can exist, increases the probability of finding environments suitable for life.Continued exploration of our solar system, particularly of Mars and the icy moons of Jupiter and Saturn, holds promise for uncovering signs of past or present microbial life. The Philosophical ImplicationsThe possibility of extraterrestrial civilizations raises profound philosophical questions about humanity’s place in the universe. Encountering another intelligence would force us to reevaluate our understanding of consciousness, culture, and ethics. It could lead to a new era of global unity as humanity comes together to face the challenges and opportunities of interstellar diplomacy. Conversely, it might also highlight our vulnerabilities and prompt introspection on our stewardship of the planet and our responsibilities as members of the cosmic community.Concluding ThoughtsWhile the existence of extraterrestrial civilizations remains a conjecture, the pursuit of answers has expanded our understanding of the universe and our place within it. The search for life beyond Earth is not just a scientific endeavor; it is a philosophical journey that challenges us to consider our origins, our destiny, and our role in the vast cosmic drama unfolding around us. Whether we find ourselves alone or part of a galactic community, the quest for knowledge about the universe and our place in it is one of humanity’s most enduring and inspiring pursuits.This essay explores various aspects of the conjectures surrounding extraterrestrial civilizations, from the scientific frameworks used to estimate their likelihood to the philosophical implications of their existence. If you have specific areas of interest within this broad topic, feel free to ask for further elaboration! If you have any further questions or need additional details on specific topics related to extraterrestrial life or astrobiology, please let me know!。
太空探索的进程、条件和意义英语作文
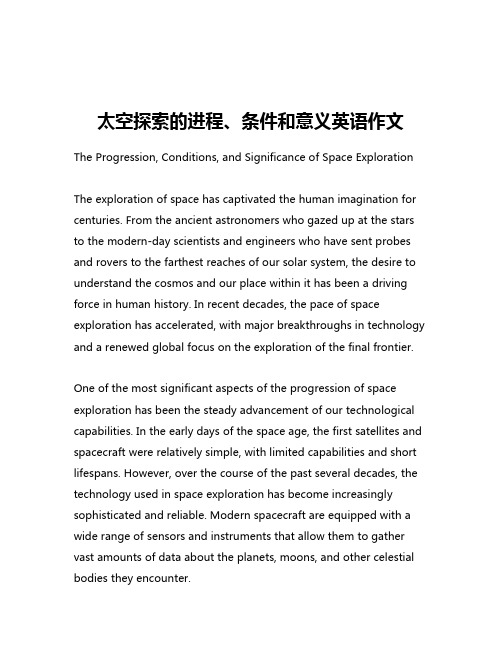
太空探索的进程、条件和意义英语作文The Progression, Conditions, and Significance of Space ExplorationThe exploration of space has captivated the human imagination for centuries. From the ancient astronomers who gazed up at the stars to the modern-day scientists and engineers who have sent probes and rovers to the farthest reaches of our solar system, the desire to understand the cosmos and our place within it has been a driving force in human history. In recent decades, the pace of space exploration has accelerated, with major breakthroughs in technology and a renewed global focus on the exploration of the final frontier.One of the most significant aspects of the progression of space exploration has been the steady advancement of our technological capabilities. In the early days of the space age, the first satellites and spacecraft were relatively simple, with limited capabilities and short lifespans. However, over the course of the past several decades, the technology used in space exploration has become increasingly sophisticated and reliable. Modern spacecraft are equipped with a wide range of sensors and instruments that allow them to gather vast amounts of data about the planets, moons, and other celestial bodies they encounter.Additionally, the development of more powerful launch vehicles has enabled the deployment of larger and more complex payloads, allowing for more ambitious and far-reaching missions. The Space Shuttle program, for example, revolutionized the way in which humans could access and work in space, while the development of reusable rocket technology by companies like SpaceX has further reduced the cost and complexity of space travel.Another key aspect of the progression of space exploration has been the growing international collaboration and cooperation that has emerged in recent years. While the early space race was largely defined by a rivalry between the United States and the Soviet Union, the modern era of space exploration has seen a much more collaborative approach, with nations and agencies from around the world working together on a wide range of projects and initiatives.The International Space Station, for example, is a shining example of this international cooperation, with astronauts and cosmonauts from a diverse array of countries working together to conduct cutting-edge research and experiments in the unique environment of low-Earth orbit. Similarly, the development of the James Webb Space Telescope, which is a joint project between NASA, the European Space Agency, and the Canadian Space Agency, is a testament to the power of global collaboration in pushing the boundaries of humanknowledge and understanding.Of course, the progression of space exploration has not been without its challenges and obstacles. The inherent risks and dangers associated with space travel, as well as the high costs and technical complexities involved, have presented significant hurdles that must be overcome. The tragic losses of the Challenger and Columbia space shuttles, for example, served as stark reminders of the dangers and uncertainties that come with pushing the boundaries of human exploration.However, despite these challenges, the continued pursuit of space exploration remains a vital and essential endeavor for humanity. The conditions necessary for successful space exploration are multifaceted and complex, requiring a combination of advanced technology, robust infrastructure, and a highly skilled and dedicated workforce.On the technological front, the development of increasingly sophisticated spacecraft, launch vehicles, and supporting systems is crucial. This includes not only the hardware and engineering that goes into the design and construction of these systems, but also the software and computer systems that are used to control and monitor them. Additionally, the continued advancement of fields like materials science, propulsion technology, and life support systemswill be essential in enabling more ambitious and long-duration space missions.In terms of infrastructure, the establishment of robust and reliable networks of ground-based facilities, such as launch pads, mission control centers, and communications hubs, is essential for supporting and coordinating space exploration activities. The development of dedicated spaceports and launch complexes, as well as the expansion of global tracking and telemetry networks, will be critical in enabling the safe and efficient operation of space missions.The human element is also a critical factor in the success of space exploration. The recruitment, training, and retention of highly skilled astronauts, engineers, scientists, and support personnel is essential for ensuring the success of space missions. This requires not only the development of specialized technical and operational skills, but also the cultivation of the physical, mental, and emotional resilience necessary to thrive in the demanding and often isolated environments of space.Ultimately, the significance of space exploration extends far beyond the immediate scientific and technological benefits that it provides. The exploration of the cosmos has the potential to unlock new frontiers of human knowledge and understanding, with the potential to yield insights that could transform our understanding of theuniverse and our place within it.Moreover, the pursuit of space exploration has the power to inspire and captivate people around the world, fostering a sense of wonder and curiosity that can drive innovation, scientific discovery, and technological progress. The iconic images of the Earth from space, for example, have had a profound impact on the way we view our planet and our shared responsibility for its stewardship.In a world faced with a growing array of complex global challenges, the continued exploration of space may also hold the key to unlocking new solutions and opportunities. The development of advanced remote sensing and monitoring technologies, for example, can help us better understand and address issues like climate change, natural resource depletion, and environmental degradation. Additionally, the potential for the exploitation of extraterrestrial resources, such as rare minerals or even renewable energy sources, could have far-reaching implications for the future of human civilization.Ultimately, the progression, conditions, and significance of space exploration are inextricably linked. The steady advancement of our technological capabilities, the growing international collaboration and cooperation, and the ongoing commitment to overcoming the challenges and obstacles that stand in our way, all contribute to thecontinued exploration and understanding of the cosmos. And as we continue to push the boundaries of human exploration, the potential rewards and benefits that await us are truly limitless.。
2022考研英语:双语阅读之NASA发现超 级地球 或存在生命篇

考研英语:双语阅读之NASA发现超级地球或存在生命篇如果要想提高我们的英语阅读能力,经常看一些双语阅读文章是一个不错的选择。
为此我整理了“考研英语:双语阅读之NASA发现"超级地球"或存在生命篇”的文章,希望对大家有所帮助。
考研英语:双语阅读之NASA发现"超级地球"或存在生命篇以下是考研英语:双语阅读之NASA发现"超级地球"或存在生命篇的具体内容:NASA发现"超级地球"或存在生命After completing its first year of observations in the southern sky, NASA's Transiting ExoplanetSurvey Satellite has spotted some intriguing new exoplanets only 31 light-years away fromEarth.宇航局的过境系外行星测量卫星(TESS)在完成对南半球天空的第一年观测后,发现了几颗距离地球仅31光年的有趣的新系外行星。
Multiple exoplanets -- planets orbiting stars outside our solar system -- were discoveredorbiting an M-dwarf star, called GJ 357 in the Hydra constellation. The star is 40% cooler thanour sun and only about a third of the sun's mass and size. A study describing the three planetswas published this week in the journal Astronomy & Astrophysics.科学家发现了数颗围绕着长蛇座M矮星GJ 357运行的系外行星,系外行星指的是围绕太阳系外恒星运行的行星。
宇宙科学潮汐锁定的英语范文

宇宙科学潮汐锁定的英语范文Tidal locking is a fascinating phenomenon in the cosmos where one side of a celestial body is perpetually facing its partner, like Earth's Moon always showing the same face to us. This occurs due to the gravitational interaction between two bodies, which slows down the rotation of the smaller oneuntil it matches the orbital period.The concept of tidal locking is not just limited to moons and planets; it's a widespread occurrence in binary star systems as well. As stars orbit each other, their mutual gravitational pull causes them to become locked in a synchronized dance, with each star rotating once for everyorbit they complete together.This locking effect has profound implications for the habitability of exoplanets. If a planet is tidally locked toits star, one side may experience perpetual daylight whilethe other is shrouded in eternal darkness. Such a stark contrast could lead to extreme temperature differences, affecting the planet's potential to support life.The study of tidal locking also helps us understand the geological and atmospheric changes on celestial bodies. For instance, the Moon's lack of a significant atmosphere ispartly due to its tidal lock with Earth, which has caused its interior to cool and solidify, preventing the generation of a magnetic field.Moreover, the search for extraterrestrial life is influenced by tidal locking. Scientists speculate that if a planet is tidally locked, life might be more likely to exist in the twilight zone, where the temperatures are moderate, and conditions are more suitable for sustaining life.Lastly, the phenomenon of tidal locking is not only a subject of scientific inquiry but also a source of inspiration for science fiction. It has led to the creation of imaginative worlds with unique day-night cycles and diverse ecosystems, sparking curiosity and wonder about the universe we inhabit.。
3GPP TS 36.331 V13.2.0 (2016-06)
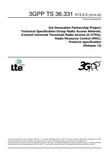
3GPP TS 36.331 V13.2.0 (2016-06)Technical Specification3rd Generation Partnership Project;Technical Specification Group Radio Access Network;Evolved Universal Terrestrial Radio Access (E-UTRA);Radio Resource Control (RRC);Protocol specification(Release 13)The present document has been developed within the 3rd Generation Partnership Project (3GPP TM) and may be further elaborated for the purposes of 3GPP. The present document has not been subject to any approval process by the 3GPP Organizational Partners and shall not be implemented.This Specification is provided for future development work within 3GPP only. The Organizational Partners accept no liability for any use of this Specification. Specifications and reports for implementation of the 3GPP TM system should be obtained via the 3GPP Organizational Partners' Publications Offices.KeywordsUMTS, radio3GPPPostal address3GPP support office address650 Route des Lucioles - Sophia AntipolisValbonne - FRANCETel.: +33 4 92 94 42 00 Fax: +33 4 93 65 47 16InternetCopyright NotificationNo part may be reproduced except as authorized by written permission.The copyright and the foregoing restriction extend to reproduction in all media.© 2016, 3GPP Organizational Partners (ARIB, ATIS, CCSA, ETSI, TSDSI, TTA, TTC).All rights reserved.UMTS™ is a Trade Mark of ETSI registered for the benefit of its members3GPP™ is a Trade Mark of ETSI registered for the benefit of its Members and of the 3GPP Organizational PartnersLTE™ is a Trade Mark of ETSI currently being registered for the benefit of its Members and of the 3GPP Organizational Partners GSM® and the GSM logo are registered and owned by the GSM AssociationBluetooth® is a Trade Mark of the Bluetooth SIG registered for the benefit of its membersContentsForeword (18)1Scope (19)2References (19)3Definitions, symbols and abbreviations (22)3.1Definitions (22)3.2Abbreviations (24)4General (27)4.1Introduction (27)4.2Architecture (28)4.2.1UE states and state transitions including inter RAT (28)4.2.2Signalling radio bearers (29)4.3Services (30)4.3.1Services provided to upper layers (30)4.3.2Services expected from lower layers (30)4.4Functions (30)5Procedures (32)5.1General (32)5.1.1Introduction (32)5.1.2General requirements (32)5.2System information (33)5.2.1Introduction (33)5.2.1.1General (33)5.2.1.2Scheduling (34)5.2.1.2a Scheduling for NB-IoT (34)5.2.1.3System information validity and notification of changes (35)5.2.1.4Indication of ETWS notification (36)5.2.1.5Indication of CMAS notification (37)5.2.1.6Notification of EAB parameters change (37)5.2.1.7Access Barring parameters change in NB-IoT (37)5.2.2System information acquisition (38)5.2.2.1General (38)5.2.2.2Initiation (38)5.2.2.3System information required by the UE (38)5.2.2.4System information acquisition by the UE (39)5.2.2.5Essential system information missing (42)5.2.2.6Actions upon reception of the MasterInformationBlock message (42)5.2.2.7Actions upon reception of the SystemInformationBlockType1 message (42)5.2.2.8Actions upon reception of SystemInformation messages (44)5.2.2.9Actions upon reception of SystemInformationBlockType2 (44)5.2.2.10Actions upon reception of SystemInformationBlockType3 (45)5.2.2.11Actions upon reception of SystemInformationBlockType4 (45)5.2.2.12Actions upon reception of SystemInformationBlockType5 (45)5.2.2.13Actions upon reception of SystemInformationBlockType6 (45)5.2.2.14Actions upon reception of SystemInformationBlockType7 (45)5.2.2.15Actions upon reception of SystemInformationBlockType8 (45)5.2.2.16Actions upon reception of SystemInformationBlockType9 (46)5.2.2.17Actions upon reception of SystemInformationBlockType10 (46)5.2.2.18Actions upon reception of SystemInformationBlockType11 (46)5.2.2.19Actions upon reception of SystemInformationBlockType12 (47)5.2.2.20Actions upon reception of SystemInformationBlockType13 (48)5.2.2.21Actions upon reception of SystemInformationBlockType14 (48)5.2.2.22Actions upon reception of SystemInformationBlockType15 (48)5.2.2.23Actions upon reception of SystemInformationBlockType16 (48)5.2.2.24Actions upon reception of SystemInformationBlockType17 (48)5.2.2.25Actions upon reception of SystemInformationBlockType18 (48)5.2.2.26Actions upon reception of SystemInformationBlockType19 (49)5.2.3Acquisition of an SI message (49)5.2.3a Acquisition of an SI message by BL UE or UE in CE or a NB-IoT UE (50)5.3Connection control (50)5.3.1Introduction (50)5.3.1.1RRC connection control (50)5.3.1.2Security (52)5.3.1.2a RN security (53)5.3.1.3Connected mode mobility (53)5.3.1.4Connection control in NB-IoT (54)5.3.2Paging (55)5.3.2.1General (55)5.3.2.2Initiation (55)5.3.2.3Reception of the Paging message by the UE (55)5.3.3RRC connection establishment (56)5.3.3.1General (56)5.3.3.1a Conditions for establishing RRC Connection for sidelink communication/ discovery (58)5.3.3.2Initiation (59)5.3.3.3Actions related to transmission of RRCConnectionRequest message (63)5.3.3.3a Actions related to transmission of RRCConnectionResumeRequest message (64)5.3.3.4Reception of the RRCConnectionSetup by the UE (64)5.3.3.4a Reception of the RRCConnectionResume by the UE (66)5.3.3.5Cell re-selection while T300, T302, T303, T305, T306, or T308 is running (68)5.3.3.6T300 expiry (68)5.3.3.7T302, T303, T305, T306, or T308 expiry or stop (69)5.3.3.8Reception of the RRCConnectionReject by the UE (70)5.3.3.9Abortion of RRC connection establishment (71)5.3.3.10Handling of SSAC related parameters (71)5.3.3.11Access barring check (72)5.3.3.12EAB check (73)5.3.3.13Access barring check for ACDC (73)5.3.3.14Access Barring check for NB-IoT (74)5.3.4Initial security activation (75)5.3.4.1General (75)5.3.4.2Initiation (76)5.3.4.3Reception of the SecurityModeCommand by the UE (76)5.3.5RRC connection reconfiguration (77)5.3.5.1General (77)5.3.5.2Initiation (77)5.3.5.3Reception of an RRCConnectionReconfiguration not including the mobilityControlInfo by theUE (77)5.3.5.4Reception of an RRCConnectionReconfiguration including the mobilityControlInfo by the UE(handover) (79)5.3.5.5Reconfiguration failure (83)5.3.5.6T304 expiry (handover failure) (83)5.3.5.7Void (84)5.3.5.7a T307 expiry (SCG change failure) (84)5.3.5.8Radio Configuration involving full configuration option (84)5.3.6Counter check (86)5.3.6.1General (86)5.3.6.2Initiation (86)5.3.6.3Reception of the CounterCheck message by the UE (86)5.3.7RRC connection re-establishment (87)5.3.7.1General (87)5.3.7.2Initiation (87)5.3.7.3Actions following cell selection while T311 is running (88)5.3.7.4Actions related to transmission of RRCConnectionReestablishmentRequest message (89)5.3.7.5Reception of the RRCConnectionReestablishment by the UE (89)5.3.7.6T311 expiry (91)5.3.7.7T301 expiry or selected cell no longer suitable (91)5.3.7.8Reception of RRCConnectionReestablishmentReject by the UE (91)5.3.8RRC connection release (92)5.3.8.1General (92)5.3.8.2Initiation (92)5.3.8.3Reception of the RRCConnectionRelease by the UE (92)5.3.8.4T320 expiry (93)5.3.9RRC connection release requested by upper layers (93)5.3.9.1General (93)5.3.9.2Initiation (93)5.3.10Radio resource configuration (93)5.3.10.0General (93)5.3.10.1SRB addition/ modification (94)5.3.10.2DRB release (95)5.3.10.3DRB addition/ modification (95)5.3.10.3a1DC specific DRB addition or reconfiguration (96)5.3.10.3a2LWA specific DRB addition or reconfiguration (98)5.3.10.3a3LWIP specific DRB addition or reconfiguration (98)5.3.10.3a SCell release (99)5.3.10.3b SCell addition/ modification (99)5.3.10.3c PSCell addition or modification (99)5.3.10.4MAC main reconfiguration (99)5.3.10.5Semi-persistent scheduling reconfiguration (100)5.3.10.6Physical channel reconfiguration (100)5.3.10.7Radio Link Failure Timers and Constants reconfiguration (101)5.3.10.8Time domain measurement resource restriction for serving cell (101)5.3.10.9Other configuration (102)5.3.10.10SCG reconfiguration (103)5.3.10.11SCG dedicated resource configuration (104)5.3.10.12Reconfiguration SCG or split DRB by drb-ToAddModList (105)5.3.10.13Neighbour cell information reconfiguration (105)5.3.10.14Void (105)5.3.10.15Sidelink dedicated configuration (105)5.3.10.16T370 expiry (106)5.3.11Radio link failure related actions (107)5.3.11.1Detection of physical layer problems in RRC_CONNECTED (107)5.3.11.2Recovery of physical layer problems (107)5.3.11.3Detection of radio link failure (107)5.3.12UE actions upon leaving RRC_CONNECTED (109)5.3.13UE actions upon PUCCH/ SRS release request (110)5.3.14Proximity indication (110)5.3.14.1General (110)5.3.14.2Initiation (111)5.3.14.3Actions related to transmission of ProximityIndication message (111)5.3.15Void (111)5.4Inter-RAT mobility (111)5.4.1Introduction (111)5.4.2Handover to E-UTRA (112)5.4.2.1General (112)5.4.2.2Initiation (112)5.4.2.3Reception of the RRCConnectionReconfiguration by the UE (112)5.4.2.4Reconfiguration failure (114)5.4.2.5T304 expiry (handover to E-UTRA failure) (114)5.4.3Mobility from E-UTRA (114)5.4.3.1General (114)5.4.3.2Initiation (115)5.4.3.3Reception of the MobilityFromEUTRACommand by the UE (115)5.4.3.4Successful completion of the mobility from E-UTRA (116)5.4.3.5Mobility from E-UTRA failure (117)5.4.4Handover from E-UTRA preparation request (CDMA2000) (117)5.4.4.1General (117)5.4.4.2Initiation (118)5.4.4.3Reception of the HandoverFromEUTRAPreparationRequest by the UE (118)5.4.5UL handover preparation transfer (CDMA2000) (118)5.4.5.1General (118)5.4.5.2Initiation (118)5.4.5.3Actions related to transmission of the ULHandoverPreparationTransfer message (119)5.4.5.4Failure to deliver the ULHandoverPreparationTransfer message (119)5.4.6Inter-RAT cell change order to E-UTRAN (119)5.4.6.1General (119)5.4.6.2Initiation (119)5.4.6.3UE fails to complete an inter-RAT cell change order (119)5.5Measurements (120)5.5.1Introduction (120)5.5.2Measurement configuration (121)5.5.2.1General (121)5.5.2.2Measurement identity removal (122)5.5.2.2a Measurement identity autonomous removal (122)5.5.2.3Measurement identity addition/ modification (123)5.5.2.4Measurement object removal (124)5.5.2.5Measurement object addition/ modification (124)5.5.2.6Reporting configuration removal (126)5.5.2.7Reporting configuration addition/ modification (127)5.5.2.8Quantity configuration (127)5.5.2.9Measurement gap configuration (127)5.5.2.10Discovery signals measurement timing configuration (128)5.5.2.11RSSI measurement timing configuration (128)5.5.3Performing measurements (128)5.5.3.1General (128)5.5.3.2Layer 3 filtering (131)5.5.4Measurement report triggering (131)5.5.4.1General (131)5.5.4.2Event A1 (Serving becomes better than threshold) (135)5.5.4.3Event A2 (Serving becomes worse than threshold) (136)5.5.4.4Event A3 (Neighbour becomes offset better than PCell/ PSCell) (136)5.5.4.5Event A4 (Neighbour becomes better than threshold) (137)5.5.4.6Event A5 (PCell/ PSCell becomes worse than threshold1 and neighbour becomes better thanthreshold2) (138)5.5.4.6a Event A6 (Neighbour becomes offset better than SCell) (139)5.5.4.7Event B1 (Inter RAT neighbour becomes better than threshold) (139)5.5.4.8Event B2 (PCell becomes worse than threshold1 and inter RAT neighbour becomes better thanthreshold2) (140)5.5.4.9Event C1 (CSI-RS resource becomes better than threshold) (141)5.5.4.10Event C2 (CSI-RS resource becomes offset better than reference CSI-RS resource) (141)5.5.4.11Event W1 (WLAN becomes better than a threshold) (142)5.5.4.12Event W2 (All WLAN inside WLAN mobility set becomes worse than threshold1 and a WLANoutside WLAN mobility set becomes better than threshold2) (142)5.5.4.13Event W3 (All WLAN inside WLAN mobility set becomes worse than a threshold) (143)5.5.5Measurement reporting (144)5.5.6Measurement related actions (148)5.5.6.1Actions upon handover and re-establishment (148)5.5.6.2Speed dependant scaling of measurement related parameters (149)5.5.7Inter-frequency RSTD measurement indication (149)5.5.7.1General (149)5.5.7.2Initiation (150)5.5.7.3Actions related to transmission of InterFreqRSTDMeasurementIndication message (150)5.6Other (150)5.6.0General (150)5.6.1DL information transfer (151)5.6.1.1General (151)5.6.1.2Initiation (151)5.6.1.3Reception of the DLInformationTransfer by the UE (151)5.6.2UL information transfer (151)5.6.2.1General (151)5.6.2.2Initiation (151)5.6.2.3Actions related to transmission of ULInformationTransfer message (152)5.6.2.4Failure to deliver ULInformationTransfer message (152)5.6.3UE capability transfer (152)5.6.3.1General (152)5.6.3.2Initiation (153)5.6.3.3Reception of the UECapabilityEnquiry by the UE (153)5.6.4CSFB to 1x Parameter transfer (157)5.6.4.1General (157)5.6.4.2Initiation (157)5.6.4.3Actions related to transmission of CSFBParametersRequestCDMA2000 message (157)5.6.4.4Reception of the CSFBParametersResponseCDMA2000 message (157)5.6.5UE Information (158)5.6.5.1General (158)5.6.5.2Initiation (158)5.6.5.3Reception of the UEInformationRequest message (158)5.6.6 Logged Measurement Configuration (159)5.6.6.1General (159)5.6.6.2Initiation (160)5.6.6.3Reception of the LoggedMeasurementConfiguration by the UE (160)5.6.6.4T330 expiry (160)5.6.7 Release of Logged Measurement Configuration (160)5.6.7.1General (160)5.6.7.2Initiation (160)5.6.8 Measurements logging (161)5.6.8.1General (161)5.6.8.2Initiation (161)5.6.9In-device coexistence indication (163)5.6.9.1General (163)5.6.9.2Initiation (164)5.6.9.3Actions related to transmission of InDeviceCoexIndication message (164)5.6.10UE Assistance Information (165)5.6.10.1General (165)5.6.10.2Initiation (166)5.6.10.3Actions related to transmission of UEAssistanceInformation message (166)5.6.11 Mobility history information (166)5.6.11.1General (166)5.6.11.2Initiation (166)5.6.12RAN-assisted WLAN interworking (167)5.6.12.1General (167)5.6.12.2Dedicated WLAN offload configuration (167)5.6.12.3WLAN offload RAN evaluation (167)5.6.12.4T350 expiry or stop (167)5.6.12.5Cell selection/ re-selection while T350 is running (168)5.6.13SCG failure information (168)5.6.13.1General (168)5.6.13.2Initiation (168)5.6.13.3Actions related to transmission of SCGFailureInformation message (168)5.6.14LTE-WLAN Aggregation (169)5.6.14.1Introduction (169)5.6.14.2Reception of LWA configuration (169)5.6.14.3Release of LWA configuration (170)5.6.15WLAN connection management (170)5.6.15.1Introduction (170)5.6.15.2WLAN connection status reporting (170)5.6.15.2.1General (170)5.6.15.2.2Initiation (171)5.6.15.2.3Actions related to transmission of WLANConnectionStatusReport message (171)5.6.15.3T351 Expiry (WLAN connection attempt timeout) (171)5.6.15.4WLAN status monitoring (171)5.6.16RAN controlled LTE-WLAN interworking (172)5.6.16.1General (172)5.6.16.2WLAN traffic steering command (172)5.6.17LTE-WLAN aggregation with IPsec tunnel (173)5.6.17.1General (173)5.7Generic error handling (174)5.7.1General (174)5.7.2ASN.1 violation or encoding error (174)5.7.3Field set to a not comprehended value (174)5.7.4Mandatory field missing (174)5.7.5Not comprehended field (176)5.8MBMS (176)5.8.1Introduction (176)5.8.1.1General (176)5.8.1.2Scheduling (176)5.8.1.3MCCH information validity and notification of changes (176)5.8.2MCCH information acquisition (178)5.8.2.1General (178)5.8.2.2Initiation (178)5.8.2.3MCCH information acquisition by the UE (178)5.8.2.4Actions upon reception of the MBSFNAreaConfiguration message (178)5.8.2.5Actions upon reception of the MBMSCountingRequest message (179)5.8.3MBMS PTM radio bearer configuration (179)5.8.3.1General (179)5.8.3.2Initiation (179)5.8.3.3MRB establishment (179)5.8.3.4MRB release (179)5.8.4MBMS Counting Procedure (179)5.8.4.1General (179)5.8.4.2Initiation (180)5.8.4.3Reception of the MBMSCountingRequest message by the UE (180)5.8.5MBMS interest indication (181)5.8.5.1General (181)5.8.5.2Initiation (181)5.8.5.3Determine MBMS frequencies of interest (182)5.8.5.4Actions related to transmission of MBMSInterestIndication message (183)5.8a SC-PTM (183)5.8a.1Introduction (183)5.8a.1.1General (183)5.8a.1.2SC-MCCH scheduling (183)5.8a.1.3SC-MCCH information validity and notification of changes (183)5.8a.1.4Procedures (184)5.8a.2SC-MCCH information acquisition (184)5.8a.2.1General (184)5.8a.2.2Initiation (184)5.8a.2.3SC-MCCH information acquisition by the UE (184)5.8a.2.4Actions upon reception of the SCPTMConfiguration message (185)5.8a.3SC-PTM radio bearer configuration (185)5.8a.3.1General (185)5.8a.3.2Initiation (185)5.8a.3.3SC-MRB establishment (185)5.8a.3.4SC-MRB release (185)5.9RN procedures (186)5.9.1RN reconfiguration (186)5.9.1.1General (186)5.9.1.2Initiation (186)5.9.1.3Reception of the RNReconfiguration by the RN (186)5.10Sidelink (186)5.10.1Introduction (186)5.10.1a Conditions for sidelink communication operation (187)5.10.2Sidelink UE information (188)5.10.2.1General (188)5.10.2.2Initiation (189)5.10.2.3Actions related to transmission of SidelinkUEInformation message (193)5.10.3Sidelink communication monitoring (195)5.10.6Sidelink discovery announcement (198)5.10.6a Sidelink discovery announcement pool selection (201)5.10.6b Sidelink discovery announcement reference carrier selection (201)5.10.7Sidelink synchronisation information transmission (202)5.10.7.1General (202)5.10.7.2Initiation (203)5.10.7.3Transmission of SLSS (204)5.10.7.4Transmission of MasterInformationBlock-SL message (205)5.10.7.5Void (206)5.10.8Sidelink synchronisation reference (206)5.10.8.1General (206)5.10.8.2Selection and reselection of synchronisation reference UE (SyncRef UE) (206)5.10.9Sidelink common control information (207)5.10.9.1General (207)5.10.9.2Actions related to reception of MasterInformationBlock-SL message (207)5.10.10Sidelink relay UE operation (207)5.10.10.1General (207)5.10.10.2AS-conditions for relay related sidelink communication transmission by sidelink relay UE (207)5.10.10.3AS-conditions for relay PS related sidelink discovery transmission by sidelink relay UE (208)5.10.10.4Sidelink relay UE threshold conditions (208)5.10.11Sidelink remote UE operation (208)5.10.11.1General (208)5.10.11.2AS-conditions for relay related sidelink communication transmission by sidelink remote UE (208)5.10.11.3AS-conditions for relay PS related sidelink discovery transmission by sidelink remote UE (209)5.10.11.4Selection and reselection of sidelink relay UE (209)5.10.11.5Sidelink remote UE threshold conditions (210)6Protocol data units, formats and parameters (tabular & ASN.1) (210)6.1General (210)6.2RRC messages (212)6.2.1General message structure (212)–EUTRA-RRC-Definitions (212)–BCCH-BCH-Message (212)–BCCH-DL-SCH-Message (212)–BCCH-DL-SCH-Message-BR (213)–MCCH-Message (213)–PCCH-Message (213)–DL-CCCH-Message (214)–DL-DCCH-Message (214)–UL-CCCH-Message (214)–UL-DCCH-Message (215)–SC-MCCH-Message (215)6.2.2Message definitions (216)–CounterCheck (216)–CounterCheckResponse (217)–CSFBParametersRequestCDMA2000 (217)–CSFBParametersResponseCDMA2000 (218)–DLInformationTransfer (218)–HandoverFromEUTRAPreparationRequest (CDMA2000) (219)–InDeviceCoexIndication (220)–InterFreqRSTDMeasurementIndication (222)–LoggedMeasurementConfiguration (223)–MasterInformationBlock (225)–MBMSCountingRequest (226)–MBMSCountingResponse (226)–MBMSInterestIndication (227)–MBSFNAreaConfiguration (228)–MeasurementReport (228)–MobilityFromEUTRACommand (229)–Paging (232)–ProximityIndication (233)–RNReconfiguration (234)–RNReconfigurationComplete (234)–RRCConnectionReconfiguration (235)–RRCConnectionReconfigurationComplete (240)–RRCConnectionReestablishment (241)–RRCConnectionReestablishmentComplete (241)–RRCConnectionReestablishmentReject (242)–RRCConnectionReestablishmentRequest (243)–RRCConnectionReject (243)–RRCConnectionRelease (244)–RRCConnectionResume (248)–RRCConnectionResumeComplete (249)–RRCConnectionResumeRequest (250)–RRCConnectionRequest (250)–RRCConnectionSetup (251)–RRCConnectionSetupComplete (252)–SCGFailureInformation (253)–SCPTMConfiguration (254)–SecurityModeCommand (255)–SecurityModeComplete (255)–SecurityModeFailure (256)–SidelinkUEInformation (256)–SystemInformation (258)–SystemInformationBlockType1 (259)–UEAssistanceInformation (264)–UECapabilityEnquiry (265)–UECapabilityInformation (266)–UEInformationRequest (267)–UEInformationResponse (267)–ULHandoverPreparationTransfer (CDMA2000) (273)–ULInformationTransfer (274)–WLANConnectionStatusReport (274)6.3RRC information elements (275)6.3.1System information blocks (275)–SystemInformationBlockType2 (275)–SystemInformationBlockType3 (279)–SystemInformationBlockType4 (282)–SystemInformationBlockType5 (283)–SystemInformationBlockType6 (287)–SystemInformationBlockType7 (289)–SystemInformationBlockType8 (290)–SystemInformationBlockType9 (295)–SystemInformationBlockType10 (295)–SystemInformationBlockType11 (296)–SystemInformationBlockType12 (297)–SystemInformationBlockType13 (297)–SystemInformationBlockType14 (298)–SystemInformationBlockType15 (298)–SystemInformationBlockType16 (299)–SystemInformationBlockType17 (300)–SystemInformationBlockType18 (301)–SystemInformationBlockType19 (301)–SystemInformationBlockType20 (304)6.3.2Radio resource control information elements (304)–AntennaInfo (304)–AntennaInfoUL (306)–CQI-ReportConfig (307)–CQI-ReportPeriodicProcExtId (314)–CrossCarrierSchedulingConfig (314)–CSI-IM-Config (315)–CSI-IM-ConfigId (315)–CSI-RS-Config (317)–CSI-RS-ConfigEMIMO (318)–CSI-RS-ConfigNZP (319)–CSI-RS-ConfigNZPId (320)–CSI-RS-ConfigZP (321)–CSI-RS-ConfigZPId (321)–DMRS-Config (321)–DRB-Identity (322)–EPDCCH-Config (322)–EIMTA-MainConfig (324)–LogicalChannelConfig (325)–LWA-Configuration (326)–LWIP-Configuration (326)–RCLWI-Configuration (327)–MAC-MainConfig (327)–P-C-AndCBSR (332)–PDCCH-ConfigSCell (333)–PDCP-Config (334)–PDSCH-Config (337)–PDSCH-RE-MappingQCL-ConfigId (339)–PHICH-Config (339)–PhysicalConfigDedicated (339)–P-Max (344)–PRACH-Config (344)–PresenceAntennaPort1 (346)–PUCCH-Config (347)–PUSCH-Config (351)–RACH-ConfigCommon (355)–RACH-ConfigDedicated (357)–RadioResourceConfigCommon (358)–RadioResourceConfigDedicated (362)–RLC-Config (367)–RLF-TimersAndConstants (369)–RN-SubframeConfig (370)–SchedulingRequestConfig (371)–SoundingRS-UL-Config (372)–SPS-Config (375)–TDD-Config (376)–TimeAlignmentTimer (377)–TPC-PDCCH-Config (377)–TunnelConfigLWIP (378)–UplinkPowerControl (379)–WLAN-Id-List (382)–WLAN-MobilityConfig (382)6.3.3Security control information elements (382)–NextHopChainingCount (382)–SecurityAlgorithmConfig (383)–ShortMAC-I (383)6.3.4Mobility control information elements (383)–AdditionalSpectrumEmission (383)–ARFCN-ValueCDMA2000 (383)–ARFCN-ValueEUTRA (384)–ARFCN-ValueGERAN (384)–ARFCN-ValueUTRA (384)–BandclassCDMA2000 (384)–BandIndicatorGERAN (385)–CarrierFreqCDMA2000 (385)–CarrierFreqGERAN (385)–CellIndexList (387)–CellReselectionPriority (387)–CellSelectionInfoCE (387)–CellReselectionSubPriority (388)–CSFB-RegistrationParam1XRTT (388)–CellGlobalIdEUTRA (389)–CellGlobalIdUTRA (389)–CellGlobalIdGERAN (390)–CellGlobalIdCDMA2000 (390)–CellSelectionInfoNFreq (391)–CSG-Identity (391)–FreqBandIndicator (391)–MobilityControlInfo (391)–MobilityParametersCDMA2000 (1xRTT) (393)–MobilityStateParameters (394)–MultiBandInfoList (394)–NS-PmaxList (394)–PhysCellId (395)–PhysCellIdRange (395)–PhysCellIdRangeUTRA-FDDList (395)–PhysCellIdCDMA2000 (396)–PhysCellIdGERAN (396)–PhysCellIdUTRA-FDD (396)–PhysCellIdUTRA-TDD (396)–PLMN-Identity (397)–PLMN-IdentityList3 (397)–PreRegistrationInfoHRPD (397)–Q-QualMin (398)–Q-RxLevMin (398)–Q-OffsetRange (398)–Q-OffsetRangeInterRAT (399)–ReselectionThreshold (399)–ReselectionThresholdQ (399)–SCellIndex (399)–ServCellIndex (400)–SpeedStateScaleFactors (400)–SystemInfoListGERAN (400)–SystemTimeInfoCDMA2000 (401)–TrackingAreaCode (401)–T-Reselection (402)–T-ReselectionEUTRA-CE (402)6.3.5Measurement information elements (402)–AllowedMeasBandwidth (402)–CSI-RSRP-Range (402)–Hysteresis (402)–LocationInfo (403)–MBSFN-RSRQ-Range (403)–MeasConfig (404)–MeasDS-Config (405)–MeasGapConfig (406)–MeasId (407)–MeasIdToAddModList (407)–MeasObjectCDMA2000 (408)–MeasObjectEUTRA (408)–MeasObjectGERAN (412)–MeasObjectId (412)–MeasObjectToAddModList (412)–MeasObjectUTRA (413)–ReportConfigEUTRA (422)–ReportConfigId (425)–ReportConfigInterRAT (425)–ReportConfigToAddModList (428)–ReportInterval (429)–RSRP-Range (429)–RSRQ-Range (430)–RSRQ-Type (430)–RS-SINR-Range (430)–RSSI-Range-r13 (431)–TimeToTrigger (431)–UL-DelayConfig (431)–WLAN-CarrierInfo (431)–WLAN-RSSI-Range (432)–WLAN-Status (432)6.3.6Other information elements (433)–AbsoluteTimeInfo (433)–AreaConfiguration (433)–C-RNTI (433)–DedicatedInfoCDMA2000 (434)–DedicatedInfoNAS (434)–FilterCoefficient (434)–LoggingDuration (434)–LoggingInterval (435)–MeasSubframePattern (435)–MMEC (435)–NeighCellConfig (435)–OtherConfig (436)–RAND-CDMA2000 (1xRTT) (437)–RAT-Type (437)–ResumeIdentity (437)–RRC-TransactionIdentifier (438)–S-TMSI (438)–TraceReference (438)–UE-CapabilityRAT-ContainerList (438)–UE-EUTRA-Capability (439)–UE-RadioPagingInfo (469)–UE-TimersAndConstants (469)–VisitedCellInfoList (470)–WLAN-OffloadConfig (470)6.3.7MBMS information elements (472)–MBMS-NotificationConfig (472)–MBMS-ServiceList (473)–MBSFN-AreaId (473)–MBSFN-AreaInfoList (473)–MBSFN-SubframeConfig (474)–PMCH-InfoList (475)6.3.7a SC-PTM information elements (476)–SC-MTCH-InfoList (476)–SCPTM-NeighbourCellList (478)6.3.8Sidelink information elements (478)–SL-CommConfig (478)–SL-CommResourcePool (479)–SL-CP-Len (480)–SL-DiscConfig (481)–SL-DiscResourcePool (483)–SL-DiscTxPowerInfo (485)–SL-GapConfig (485)。
Exploring the Boundaries of Space

Exploring the Boundaries of Space Exploring the boundaries of space is an endeavor that has captivated the human imagination for centuries. The idea of venturing beyond our own planet and into the vast expanse of the universe has inspired countless scientific discoveries, technological advancements, and works of art and literature. However, the exploration of space also presents a myriad of challenges and ethical considerations that must be carefully navigated. From a scientific perspective, the exploration of space holds immense potential for expanding our understanding of the universe and our place within it. Through endeavors such as the Hubble Space Telescope and the Mars rover missions, scientists have been able to gather valuable data about distant galaxies, planetary bodies, and the conditions of outer space. This information has not only deepened our knowledge of astrophysics and cosmology but has also contributed to practical applications such as satellite technology and space travel. On the other hand, the exploration of space also raises ethical and philosophical questions about the impact of human activities beyond Earth. As we venture further into space, we must consider the potential consequences of our presence on other celestial bodies and the delicate ecosystems they may harbor. Additionally, the prospect of encountering extraterrestrial life forms raises complex ethical dilemmas about our responsibilities as stewards of the universe and the potential implications of contact with other intelligent beings. Furthermore, the exploration of space is also deeply intertwined with political and economic considerations. Space agencies and private companies around the world are engaged in a race to develop the technology and infrastructure necessary for space exploration, driven by a combination of national pride, scientific ambition, and commercial interests. This competition has led to collaborations and rivalries between nations, as well as debates about the allocation of resources and the prioritization of space exploration in relation to other pressing global issues. From a cultural and artistic perspective, the exploration of space has inspired countless works of literature, film, and visual art that reflect humanity's fascination with the unknown. Whether through the imaginative worlds of science fiction or the awe-inspiring images captured by space probes and telescopes, the exploration of space has sparked the creativeimagination and encouraged contemplation of the profound mysteries of the cosmos. On a personal level, the exploration of space evokes a sense of wonder and curiosity about the possibilities that lie beyond our own planet. The idea of venturing into the unknown and experiencing the beauty and grandeur of the universe firsthand is a source of inspiration and motivation for many individuals, driving them to pursue careers in science, engineering, and space exploration. In conclusion, the exploration of space encompasses a wide range of scientific, ethical, political, and cultural considerations that reflect the complexity of human endeavors beyond Earth. As we continue to push the boundaries of space exploration, it is essential to approach these challenges with a thoughtful and holistic perspective that takes into account the potential benefits and risks of our actions. Ultimately, the exploration of space offers a profound opportunity to expand our knowledge, inspire our imagination, and cultivate a deeper appreciation for the interconnectedness of all life within the cosmos.。
Space Exploration

Space ExplorationThe exploration of space has been an ongoing endeavor for humanity since the dawn of time. From the early days of stargazing to the modern era of space exploration, humans have always been fascinated with the mysteries of the universe beyond our planet. The exploration of space has led to many technological advancements and scientific discoveries that have changed the way we live our lives.One of the most significant accomplishments of space exploration is the development of satellite technology. Satellites have revolutionized our world by providing us with instant access to information from all corners of the globe. They have also made possible the use of GPS systems, which have become essential in our daily lives. Satellites have also played a crucial role in weather forecasting, disaster relief efforts, and national security. Another significant achievement of space exploration is the landing of humans on the moon. On July 20th, 1969, Neil Armstrong became the first person to step foot on the moon as part of NASA's Apollo 11 mission. This historic event was a testament to human ingenuity and determination. It served as a source of inspiration for generations to come and paved the way for further exploration of space.Space exploration has also led to advances in medicine. The research conducted in space has allowed for a better understanding of the human body's response to zero-gravity environments. This research has led to the development of new treatments for conditions such as osteoporosis and muscle atrophy. Additionally, the experiments conducted in space have helped scientists discover new ways to fight diseases such as cancer. Moreover, space exploration has led to the discovery of new worlds beyond our solar system. With the help of advanced telescopes and other technologies, astronomers have discovered thousands of exoplanets orbiting distant stars. These discoveries have expanded our understanding of the universe and provided new insights into the possibility of life beyond Earth.In conclusion, space exploration has been one of humanity's greatest achievements. It has led to significant advancements in technology, science, and medicine. The exploration of space has broadened our understanding of the universe, and it has inspired us to push the boundaries of what is possible. As we continue to explore the cosmos, we will undoubtedly discover new wonders and mysteries that will shape the course of human history.。
Spotlight SAR data focusing based on a two-step processing approach
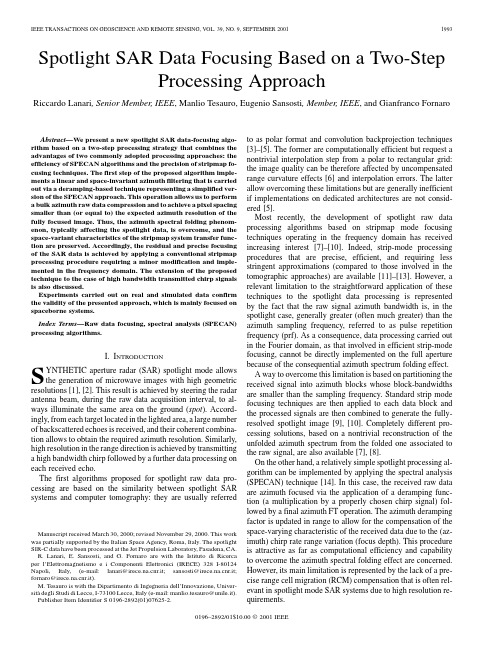
Spotlight SAR Data Focusing Based on a Two-StepProcessing ApproachRiccardo Lanari,Senior Member,IEEE,Manlio Tesauro,Eugenio Sansosti,Member,IEEE,and Gianfranco FornaroAbstract—We present a new spotlight SAR data-focusing algo-rithm based on a two-step processing strategy that combines the advantages of two commonly adopted processing approaches:the efficiency of SPECAN algorithms and the precision of stripmap fo-cusing techniques.The first step of the proposed algorithm imple-ments a linear and space-invariant azimuth filtering that is carried out via a deramping-based technique representing a simplified ver-sion of the SPECAN approach.This operation allows us to perform a bulk azimuth raw data compression and to achieve a pixel spacing smaller than(or equal to)the expected azimuth resolution of the fully focused image.Thus,the azimuth spectral folding phenom-enon,typically affecting the spotlight data,is overcome,and the space-variant characteristics of the stripmap system transfer func-tion are preserved.Accordingly,the residual and precise focusing of the SAR data is achieved by applying a conventional stripmap processing procedure requiring a minor modification and imple-mented in the frequency domain.The extension of the proposed technique to the case of high bandwidth transmitted chirp signals is also discussed.Experiments carried out on real and simulated data confirm the validity of the presented approach,which is mainly focused on spaceborne systems.Index Terms—Raw data focusing,spectral analysis(SPECAN) processing algorithms.I.I NTRODUCTIONS YNTHETIC aperture radar(SAR)spotlight mode allows the generation of microwave images with high geometric resolutions[1],[2].This result is achieved by steering the radar antenna beam,during the raw data acquisition interval,to al-ways illuminate the same area on the ground(spot).Accord-ingly,from each target located in the lighted area,a large number of backscattered echoes is received,and their coherent combina-tion allows to obtain the required azimuth resolution.Similarly, high resolution in the range direction is achieved by transmitting a high bandwidth chirp followed by a further data processing on each received echo.The first algorithms proposed for spotlight raw data pro-cessing are based on the similarity between spotlight SAR systems and computer tomography:they are usually referredManuscript received March30,2000;revised November29,2000.This work was partially supported by the Italian Space Agency,Roma,Italy.The spotlight SIR-C data have been processed at the Jet Propulsion Laboratory,Pasadena,CA. nari,E.Sansosti,and G.Fornaro are with the Istituto di Ricerca per l’Elettromagnetismo e i Componenti Elettronici(IRECE)328I-80124 Napoli,Italy,(e-mail:lanari@r.it;sansosti@r.it; fornaro@r.it).M.Tesauro is with the Dipartimento di Ingegneria dell’Innovazione,Univer-sitàdegli Studi di Lecce,I-73100Lecce,Italy(e-mail:manlio.tesauro@unile.it). Publisher Item Identifier S0196-2892(01)07625-2.to as polar format and convolution backprojection techniques [3]–[5].The former are computationally efficient but request a nontrivial interpolation step from a polar to rectangular grid: the image quality can be therefore affected by uncompensated range curvature effects[6]and interpolation errors.The latter allow overcoming these limitations but are generally inefficient if implementations on dedicated architectures are not consid-ered[5].Most recently,the development of spotlight raw data processing algorithms based on stripmap mode focusing techniques operating in the frequency domain has received increasing interest[7]–[10].Indeed,strip-mode processing procedures that are precise,efficient,and requiring less stringent approximations(compared to those involved in the tomographic approaches)are available[11]–[13].However,a relevant limitation to the straightforward application of these techniques to the spotlight data processing is represented by the fact that the raw signal azimuth bandwidth is,in the spotlight case,generally greater(often much greater)than the azimuth sampling frequency,referred to as pulse repetition frequency(prf).As a consequence,data processing carried out in the Fourier domain,as that involved in efficient strip-mode focusing,cannot be directly implemented on the full aperture because of the consequential azimuth spectrum folding effect.A way to overcome this limitation is based on partitioning the received signal into azimuth blocks whose block-bandwidths are smaller than the sampling frequency.Standard strip mode focusing techniques are then applied to each data block and the processed signals are then combined to generate the fully-resolved spotlight image[9],[10].Completely different pro-cessing solutions,based on a nontrivial reconstruction of the unfolded azimuth spectrum from the folded one associated to the raw signal,are also available[7],[8].On the other hand,a relatively simple spotlight processing al-gorithm can be implemented by applying the spectral analysis (SPECAN)technique[14].In this case,the received raw data are azimuth focused via the application of a deramping func-tion(a multiplication by a properly chosen chirp signal)fol-lowed by a final azimuth FT operation.The azimuth deramping factor is updated in range to allow for the compensation of the space-varying characteristic of the received data due to the(az-imuth)chirp rate range variation(focus depth).This procedure is attractive as far as computational efficiency and capability to overcome the azimuth spectral folding effect are concerned. However,its main limitation is represented by the lack of a pre-cise range cell migration(RCM)compensation that is often rel-evant in spotlight mode SAR systems due to high resolution re-quirements.0196–2892/01$10.00©2001IEEEIn this paper,we propose an alternative spotlight data fo-cusing technique based on decoupling the overall focusing oper-ation in two main steps.The key point of the proposed approach is to combine the advantages of efficient SPECAN and precise stripmap focusing approaches.In particular,the first processing step carries out a filtering operation aimed to achieve a bulk az-imuth raw data compression and an output pixel spacing smaller than(or equal to)the expected final azimuth resolution.Similar to SPECAN processing algorithms,this filtering operation is ef-ficiently carried out via a deramping-based approach[14]but, at variance of the former,the chirp rate of the deramping func-tion is kept constant and properly fixed at a convenient value. This is a key point in the proposed processing procedure that al-lows preserving the space variant characteristic of the residual system transfer function(STF).A discussion on the impact of the chirp rate selection on possible artifacts that may appear at the image borders is also provided.The second processing step carries out the residual focusing of the data via the use of a conventional stripmap processing pro-cedure implemented in the frequency domain and requiring only minor modifications in the available codes.This spectral do-main focusing operation is now possible because,following the bulk azimuth compression,the folding effect of the raw signal azimuth spectrum has been totally overcome.More precisely, this second(residual)processing step performs the precise RCM compensation,the data range compression and the residual az-imuth data compression;the latter accounts for higher order terms not compensated in the bulk azimuth processing step.The minor modifications to be performed in available stripmap pro-cessing codes are essentially a change of the azimuth filter func-tion,which accounts for the already compensated quadratic az-imuth phase term,and a change in the azimuth pixel spacing of the input data.It is worth noting the role that the bulk azimuth compres-sion operation plays in our approach to a preprocessing step that extends the processing capability of conventional stripmap fo-cusing procedures to spotlight data.In addition,the proposed processing algorithm does not require a specific manipulation and/or interpolation of the data,such as those necessary in az-imuth block divisions or in unfolded signal spectrum reconstruc-tion-based algorithms.Accordingly,we have finally achieved a processing procedure that is simple,precise and computation-ally efficient because it does not imply any significant increase of the raw data matrix dimensions and only includes fast Fourier transforms(FFTs)and matrix multiplication.Moreover,it can be easily extended to the case of high bandwidth transmitted sig-nals wherein spectral folding effects could appear in the range direction as well.In our case,the implemented solution is based again on a deramping approach,that is,at variance of conven-tional focusing techniques performed following the A/D con-version rather than before.A number of experiments carried out on a simulated and a real data set,the latter acquired by the experimental C-band sensor of the SIR-C system during the SIR-C/X-SAR mission in1994[9],demonstrate the validity of the presented approach.As a final remark,we want to stress that the presented anal-ysis is focused on spaceborne systems typically characterized by small squint angles[15]during the acquisition(often lessthan Fig.1.Spotlight system geometry.1-axis,assumed coincident with the platform flight path,is referred to as azimuthdirection are the(closest approach)target range and look angle,respectively.1We assume in the following that the sensor,mounted onboard a platform moving at the constantvelocity,transmits,attimes(1) whereangular carrierfrequency;chirp rate,beingis the systemwavelength,,the two-way antenna pattern factor,and being the azimuth dimension of the real,onboard antenna.Note that the assumed simplificationon allows avoiding the antenna footprint dependence on the platform location whose impact is 1Note that we have assumed the platform trajectory to be a straight line which is appropriate for airborne but not for spaceborne sensors.However,it can be shown that spaceborne data can be processed in the same manner as airborne data if the closed approach distance and the azimuth velocity are properly con-sidered[16]or,more precisely,via the appropriate sensor-target distance eval-uation[17].LANARI et al.:SPOTLIGHT SAR DATA FOCUSING 1995inessential for the following analysis.A more detailed discus-sion on this matter can be found in [10].Let us now consider a pointtargetandreceived onboard is represented,after the heterodyne process [that removes the fast varyingterm(4a)FTis the azimuth (spatial)frequency.Equation (7)shows that theazimuth spectrum is centered on thefrequencyand that the signal bandwidthiswith respect to the strip mode case,for whichit wouldbe.In this case,weget(8)Since the maximum valueof,i.e.,that relative to the nearest range,should be con-sidered.This is assumed hereafter,although we underline that in the spotlight case,due to the typically limited range extension of the illuminated spot,the range dependenceof (9)in order to avoid any azimuth spectral folding effect [18].On the other hand,this sampling frequency increase would lead to large data rates and could generate severe range ambiguity problems [15].Accordingly,the valuesofand are the raw data and thefocused image azimuth pixel dimensions,respectively,the latter chosen in agreement with the Nyquist limit available from (8).In this case,we get from(9)(10)1996IEEE TRANSACTIONS ON GEOSCIENCE AND REMOTE SENSING,VOL.39,NO.9,SEPTEMBER2001 Equation(10)clarifies that the azimuth number of pixel inthe raw data set and in the focused image,i.e.,,respectively,are comparable,and they become closeras.III.B ULK A ZIMUTH R AW D ATA C OMPRESSIONLet us now investigate a possible solution to the azimuth spec-tral folding effect discussed in the previous section.The pro-posed approach is based on a linear and space-invariant azimuthfiltering operation that performs a bulk azimuth data compres-sion and achieves an output pixel spacing,satisfying the Nyquistlimit shown in(8).This operation is efficiently implementedwithout any large zero padding step,via a deramping-basedtechnique[1],[2],[14].A.Continuous Domain AnalysisKey point of the presented technique is the azimuth convolu-tion between the raw data and the quadratic phasesignal(11)whereinand are the nearest and the farthest ranges of theilluminated spot,respectively,and represents the range valueof a generic point target located within the spot.No specific as-sumption has yet been made on the factor in(11),althoughwe anticipate that the impact of any particular selection for thisterm is later discussed in detail.We also underline that the rawsignal range component,accounting for the range independent(RI)and range dependent(RD)RCM effects,is neglected inthe azimuth convolution operation presented in this section.Allthese components are restored and accounted for during the sub-sequent and highly precise second processing step.The azimuth convolution between thesignal in(5),forthe case of an isolated target,and thefunction in(11)gives(12)wherein thesymbolof the target and on the valueof.The second line in(12)shows that this azimuth convo-lution is essentially a deramping based(SPECAN)processing,involving a chirp multiplication of the azimuth signal,a subse-quent FT and a residual phase cancellation.Indeed,but for theabove mentioned approximations,this processing step allows usto achieve an azimuth compression which is full only for thosetargets locatedat.This point can be clarified by recon-sidering(12).Indeed,if weassume(13)wherein the imaged target is fully azimuth focused.Forany,by assuming the validity of theSPM method3weget,for which the resulting signal is centeredaround andextendsfor.However,because the range ex-tension of the spot area is typically very small,we canassumeand,even in this limiting case,a compression effect,althoughpartial with respect to that achieved in(13),is obtained.The obtained results apply to the case of an isolated target,however they can be easily extended to the case of an illumi-nated area.Accounting for the azimuth spotdimensionwith(16)3Generalization to those cases where SPM cannot be applied can be derivedas in[19].Here we are interested only in having a rough measure of the targetecho extension following the first processing step.LANARI et al.:SPOTLIGHT SAR DATA FOCUSING 1997with ,i.e.,with a pixel spacing satisfying the Nyquist limit of the spotlight signal,see (8)and (9).Accordingly,sim-ilarly to what is shown in the continuous analysis presented in the previous section,(12)becomesbeing the nearest integer operator.Note that,dueto(10),with (18)where thefactorrepresents the output azimuth data repli-cation.Accordingly,under the validity of the inequality in (18),not only the azimuth spectral folding effects are avoided,see (16),but also no data wrap around occurs in the azimuth direc-tion.We note that the validity of the aforementioned inequality in (18)is generally satisfied due to the presence of a slight az-imuth data oversampling carried out on the spotlight signal with respect to the Nyquist rate that we would have with the system operating in the stripmap mode.This point can be clarified by accounting for (15)in the inequality in (18).In this case,wegetgives a value ofaboutfor the right-hand side factor in (21).This leads tothe newinequality,which is satisfied for most real spotlight SAR systems.Of course in the (rare)case of an insufficient oversampling factor,a balancing choice would be represented by setting at the midrange swath,thus leading to a resolution degradation at the image near and far range edges.Anyway,we remark that this is generally not a very critical issue because,due to the antenna beam steering,those targets would be in any case characterized by a lower resolution [10].Based on (18),we can finally rewrite (17)asfollows:of the orderofis required and implemented via the substitu-tionin (11)[and equivalently in (17)]to compen-sate for this effect.Secondly,due to the appearance of a sig-nificant range walk effect [15]in the RCM,an additional edge degradation could appear even at midrange.Although the paper is focused on low squint angle acquisitions,we stress that well known procedures applied for mitigating the range walk effect in deramping-based focusing approaches could be considered [20].However,this is worth pursuing for future studies.4Notealso that,at variance with what is shown in (22),a more conventionalexpression of the DFT operation can be consideredimplying1with n =0P=2;...;P=201[18].Inthis case,a trivial manipulation of (22)is required.1998IEEE TRANSACTIONS ON GEOSCIENCE AND REMOTE SENSING,VOL.39,NO.9,SEPTEMBER2001 IV.R ESIDUAL D ATA F OCUSING VIA S TRIPMAPP ROCESSING T ECHNIQUESLet us concentrate on the result of the bulk azimuth com-pression step shown in(12).We underline that,following thisoperation,the folding effect influencing the azimuth spectrumis avoided and the space-variant characteristics of the systemtransfer function are maintained.Accordingly,it is possible tocarry out the residual focusing of the data via the use of effi-cient and precise techniques originally designed for stripmapSAR data focusing that are implemented in the frequency do-main.To clarify this point,we refer to the expression of the receivedsignal over a distributed scene by resorting the linearity of thesystemrepresents the reflectivity function of the illumi-nated scene including the fast varying phase term in(24).Thereceived data spectrum can be written asfollows:is the range(spatial)frequency,andcan be found via the application ofthe SPM,leading to the following expression[15]:(27)where,and.In this case,wehaveFT(29)wherein the phasefactor accounts for thebulk compression step.By finally substituting(27)in(29),weget(30)withinsteadof[15].Moreover,the folding effects influencing the azimuth raw signalspectrum(see Section II)have been avoided due to the alreadycarried out bulk azimuth compression step leading to the newpixelspacing shown in(18).We also note that the space-variant characteristics of the system transfer function are pre-served by the bulk compression.This nonlinear mapping of therange frequencies,i.e.,bysimply accounting for the system transfer functioncomponentinsteadof and by considering the new azimuth sam-pling frequency.In particular,we have considered the stripmap processing ap-proach described in[12],and the overall processing block dia-gram is shown in Fig.2.In this case,the first step carries out thebulk azimuth compression,while the residual focusing is im-plemented as follows:the filtering operation,carried out in thetwo-dimensional(2-D)frequency domain via the filterfunctionallows us to fully focus the midspot area by accountingLANARI et al.:SPOTLIGHT SAR DATA FOCUSING1999Fig. 2.Two-step focusing procedure block diagram.Note that i=0P=2;...;P=201and l=0M=2;...;M=201.Moreover,1=(P1x)and1=(M1ri i in i l i l inr r i l lo re i rara r l l i in i ir i ra r i i ira r i r i im l inis r i i la r in l ii r i l r if l id i x r ii r r ic l x r i re ii i2000IEEE TRANSACTIONS ON GEOSCIENCE AND REMOTE SENSING,VOL.39,NO.9,SEPTEMBER2001 Fig.4.C-band VV-polarized image of the Sidney zone obtained by applyingthe focusing approach of Fig.5to the raw data set acquired in1994by theSIR-C system operating in an experimental spotlight mode.The expectedazimuth resolution is about1m,but the image is represented with an azimuthpixel spacing of about6.5m to avoid the geometric distortions caused bydifferent dimensions of the pixel in range and azimuth directions.The extensionof the area is of about1.7km24.5km.range compressionof(zeropadded to increase its extensionfrom)and thesignalin(31),becomingLANARI et al.:SPOTLIGHT SAR DATA FOCUSING2001Fig.5.Simulated image obtained after the bulk azimuth compression(rangecompression has been also implemented).The range corresponding to~r ishighlighted.Clearly,although not explicitly mentioned,the range pixelspacing resulting from the range focusing operation of(33)must also be considered for the implementation of theresidual focusing step.As final remarks,we underline that all the operations involvedin(33)are assumed,in our case,to be carried out after the A/Dconversion in the receiver.Moreover,the computational effi-ciency of the procedure in Fig.3can be further improved bycombining the range compression operation and the compen-sation of the scaling factor2002IEEE TRANSACTIONS ON GEOSCIENCE AND REMOTE SENSING,VOL.39,NO.9,SEPTEMBER 2001TABLE IIR ESULTSOF THEA NALYSIS C ARRIED O UT ONTHE I MAGED P OINTT ARGETS OF F IG .61994by the C-band sensor of the SIR-C system operated in the experimental spotlight mode (see Table I for a description of the system parameters).In this case,because of theratiokm,andthe near,mid,and far range distancesarekm,km,and km,respectively.Theselected value ofiskm.Accordingly,based on the analysis of Section III,we can evaluate the minimum andmaximum range distance,forexampleand ,which ensure the absence of degradation at the edges of the image,by using (20).They are givenbyKmand(36)thus guaranteeing the possibility of focusing the overall scene.The image obtained by applying the procedure of Fig.2is pre-sented in Fig.4.It clearly shows the focusing capability of the proposed algorithm.However,the absence of known reference targets in the scene does not allow any significant quantitative measurement of the quality of the obtained image.Accordingly,in order to assess the performance of the proposed approach,we have generated a simulated data set representing the signal backscattered by a sequence of three point targets aligned in the range direction and located over an absorbing background.The system parameters are again those of Table I.To better clarify the effect of the bulk azimuth compression step,we show the result obtained by applying this operation (see Fig.5).As expected,the achieved azimuth compression 5effect is more relevant for the target located at a range closer to .We additionally remark that the azimuth extension of the bulk com-pressed data is of 2048samples,and it has been increased 6with respect to the raw data,by about 20%(the azimuth raw data length was of 1700samples),but no additional data dimension increase is required in the residual focusing step.Note also in5Inorder to improve the readability of the result,a range compression step has been also carried out.6This allowed the use of high efficient FFT codes with a power of two data lengths[18].Fig.7.High resolution simulated image obtained by applying the focusing procedure of Fig.3.The contour plots of the three imaged point targets are also shown.Fig.5the effect of the uncompensated range cell migration ef-fect.The fully focused image is finally shown in Fig.6.The results of the measurements carried out on the imaged point targets of Fig.6are summarized in Table II wherein the theoretical az-imuth resolution values are those pertinent to the selected point reflector.The inspection of Table II clarifies the high perfor-mance of the presented technique for what concerns the ampli-tude characteristics of the target responses.The phase accuracy has been also assessed;it is about 1LANARI et al.:SPOTLIGHT SAR DATA FOCUSING2003 TABLE IIIR ESULTS OF THE A NALYSIS C ARRIED O UT ON THE I MAGED P OINTT ARGETS OF F IG.712004IEEE TRANSACTIONS ON GEOSCIENCE AND REMOTE SENSING,VOL.39,NO.9,SEPTEMBER 2001different foreign research institutes such as the Institute of Space and Astronau-tical Science (ISAS),Tokyo,Japan,the German Aerospace Research Establish-ment (DLR),Oberpfafenhoffen,Germany,and the Jet Propulsion Laboratory (JPL),Pasadena,CA,where he received a NASA recognition for the innovative development of a ScanSAR processor for the SRTM mission.His main research activities are in the SAR data processing field as well as in IFSAR techniques.On this topic,he has authored 30international journal papers and,more recently,a book Synthetic Aperture Radar Processing (Boca Raton,FL:CRC).He also holds two patents on SAR raw data processing techniques.nari has been Chairman at several international conferences and was invited to join the technical program committee for the IGARSS Conference in 2000and2001.Manlio Tesauro received the Laurea degree (summa cum laude)in electronic engineering and the Ph.D.degree in electronic engineering and computer sci-ence,both from the University of Napoli “Federico II,”Napoli,Italy,in 1992and 1998,respectively.In 1998and 1999,he was with the Istituto di Ricerca per l’Elettromagnetismo ed I Componenti Elettronici (IRECE),Napoli,National Research Council (CNR),with a grant from Telespazio.Since 2000,he has been a Research Scientist with the Dipartimento di Ingegneria dell’Innovazione,University of Lecce,Lecce,Italy.In February 2000,he was a member of the Italian Team in the ASI Ground Data Processing Chain during the Shuttle Radar Topography Mission (SRTM)at the Jet Propulsion Laboratory,Pasadena,CA.His main interests are in the field of statistical signal processing with emphasis on SAR and IFSARprocessing.Eugenio Sansosti (M’96)received the Laurea degree (summa cum laude)in electronic engineering from the University of Napoli “Federico II,”Napoli,Italy,in 1995.Since 1997,he has been with the Istituto di Ricerca per l’Elettromagnetismo e I Componenti Elettronici (IRECE),National Research Council (CNR),where he currently holds a Full Researcher position.He is also an Adjunct Professor of electrical Communica-tions at the University of Cassino,Cassino,Italy.He was a Guest Scientist with the Jet Propulsion Labora-tory,Pasadena,CA,from August 1997to February 1998,and again in February 2000in support of the NASA Shuttle Radar Topography Mission.In November and December 2000,he worked as an Image Processing Adviser at the Istituto Tecnologico de Aeronautica (ITA),Sao Josédos Campos SP,Brazil.His main research interests are in airborne and spaceborne synthetic aperture radar (SAR)data processing,SAR interferometry,and differential SARinterferometry.Gianfranco Fornaro received the Laurea degree in electronic engineering from the University of Napoli “Federico II,”Napoli,Italy,in 1992,and the Ph.D.degree from the University of Rome “La Sapienza,”Rome,Italy,in 1997.He is currently a Full Researcher at the Istituto di Ricerca per l’Elettromagnetismo e i Componenti Elettronici (IRECE),Italian National Research Council (CNR)and Adjunct Professor of Communi-cation,University of Cassino,Cassino,Italy.He has been a Visiting Scientist with the German AerospaceEstablishment (DLR),Oberpfafenhoffen,Germany,and the Politecnico de Milano,Milano,Italy,and has been a Lecturer with the Istituto Tecnologico de Aeronautica (ITA),Sao Josédos Campos SP,Brasil.His main research interests are in the signal processing field with applications to the synthetic aperture radar (SAR)data processing,SAR interferometry,and differential SAR interferometry.Dr.Fornaro was awarded the Mountbatten Premium Award by the Institution of Electrical Engineers (IEE)in 1997.。
法布里珀罗基模共振英文

法布里珀罗基模共振英文The Fabryperot ResonanceOptics, the study of light and its properties, has been a subject of fascination for scientists and researchers for centuries. One of the fundamental phenomena in optics is the Fabry-Perot resonance, named after the French physicists Charles Fabry and Alfred Perot, who first described it in the late 19th century. This resonance effect has numerous applications in various fields, ranging from telecommunications to quantum physics, and its understanding is crucial in the development of advanced optical technologies.The Fabry-Perot resonance occurs when light is reflected multiple times between two parallel, partially reflective surfaces, known as mirrors. This creates a standing wave pattern within the cavity formed by the mirrors, where the light waves interfere constructively and destructively to produce a series of sharp peaks and valleys in the transmitted and reflected light intensity. The specific wavelengths at which the constructive interference occurs are known as the resonant wavelengths of the Fabry-Perot cavity.The resonant wavelengths of a Fabry-Perot cavity are determined bythe distance between the mirrors, the refractive index of the material within the cavity, and the wavelength of the incident light. When the optical path length, which is the product of the refractive index and the physical distance between the mirrors, is an integer multiple of the wavelength of the incident light, the light waves interfere constructively, resulting in a high-intensity transmission through the cavity. Conversely, when the optical path length is not an integer multiple of the wavelength, the light waves interfere destructively, leading to a low-intensity transmission.The sharpness of the resonant peaks in a Fabry-Perot cavity is determined by the reflectivity of the mirrors. Highly reflective mirrors result in a higher finesse, which is a measure of the ratio of the spacing between the resonant peaks to their width. This high finesse allows for the creation of narrow-linewidth, high-resolution optical filters and laser cavities, which are essential components in various optical systems.One of the key applications of the Fabry-Perot resonance is in the field of optical telecommunications. Fiber-optic communication systems often utilize Fabry-Perot filters to select specific wavelength channels for data transmission, enabling the efficient use of the available bandwidth in fiber-optic networks. These filters can be tuned by adjusting the mirror separation or the refractive index of the cavity, allowing for dynamic wavelength selection andreconfiguration of the communication system.Another important application of the Fabry-Perot resonance is in the field of laser technology. Fabry-Perot cavities are commonly used as the optical resonator in various types of lasers, providing the necessary feedback to sustain the lasing process. The high finesse of the Fabry-Perot cavity allows for the generation of highly monochromatic and coherent light, which is crucial for applications such as spectroscopy, interferometry, and precision metrology.In the realm of quantum physics, the Fabry-Perot resonance plays a crucial role in the study of cavity quantum electrodynamics (cQED). In cQED, atoms or other quantum systems are placed inside a Fabry-Perot cavity, where the strong interaction between the atoms and the confined electromagnetic field can lead to the observation of fascinating quantum phenomena, such as the Purcell effect, vacuum Rabi oscillations, and the generation of nonclassical states of light.Furthermore, the Fabry-Perot resonance has found applications in the field of optical sensing, where it is used to detect small changes in physical parameters, such as displacement, pressure, or temperature. The high sensitivity and stability of Fabry-Perot interferometers make them valuable tools in various sensing and measurement applications, ranging from seismic monitoring to the detection of gravitational waves.The Fabry-Perot resonance is a fundamental concept in optics that has enabled the development of numerous advanced optical technologies. Its versatility and importance in various fields of science and engineering have made it a subject of continuous research and innovation. As the field of optics continues to advance, the Fabry-Perot resonance will undoubtedly play an increasingly crucial role in shaping the future of optical systems and applications.。
剑8 test 3 reading passage 1
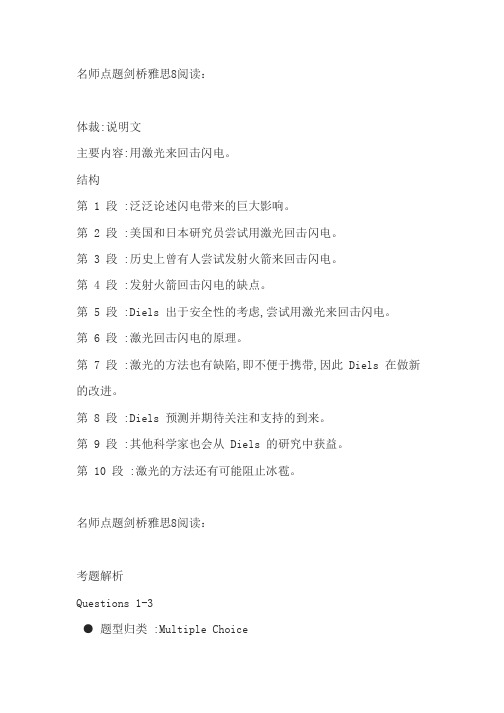
名师点题剑桥雅思8阅读:体裁:说明文主要内容:用激光来回击闪电。
结构第1 段:泛泛论述闪电带来的巨大影响。
第2 段:美国和日本研究员尝试用激光回击闪电。
第3 段:历史上曾有人尝试发射火箭来回击闪电。
第4 段:发射火箭回击闪电的缺点。
第5 段:Diels 出于安全性的考虑,尝试用激光来回击闪电。
第6 段:激光回击闪电的原理。
第7 段:激光的方法也有缺陷,即不便于携带,因此Diels 在做新的改进。
第8 段:Diels 预测并期待关注和支持的到来。
第9 段:其他科学家也会从Diels 的研究中获益。
第10 段:激光的方法还有可能阻止冰雹。
名师点题剑桥雅思8阅读:考题解析Questions 1-3 ●题型归类:Multiple Choice题目解析名师点题剑桥雅思8阅读:考题解析 Questions 4-6 ●题型归类:Sentence Completion 题目解析名师点题剑桥雅思8阅读:Questions 7-10●题型归类:Summary题目解析名师点题剑桥雅思8阅读:考题解析 Questions 11-13 ●题型归类:YES/NO/NOT GIVEN 题目解析名师点题剑桥雅思8阅读:参考译文用激光来回击闪电在所有的天气中,几乎没有比大雷雨侵袭时更为猛烈的了。
每年,仅仅在美国,猛烈的大雷雨电流就造成大约500人伤亡。
当阴云滚滚而来时,野外休闲的高尔夫场地可能会成为令人惶恐的危险之地,孤身一人的高尔夫球手或许会成为闪电之箭最有吸引力的目标。
另外,它还会造成财产损失。
美国电力公司每年遭受的闪电损失就超过1亿美元。
然而,美国和日本的研究人员正在谋划应对措施。
他们已经在实验中试验了一些将大雷雨能量中性化的策略,并且,他们将于今年冬天直面真实的暴风雨,他们将装备一库房的激光,这些激光将指向天空,在闪电袭击前就将雷雨云的电量释放掉。
让暴雨云乖乖地释放闪电的这个想法并不新鲜。
在20世纪60年代初,研究人员尝试向雷雨云中发射火箭跟踪电线,以建立一条便捷的路径,使这些云层产生的巨大电荷得以释放。
clarify汇总

Sliding Spotlight SAR1Converse Beam Cross Sliding Spotlight SAR2TerraSAR-X,New Formulation of the Extended Chirp Scaling Algorithm3Hybrid Bistatic(双基地),in the Double Sliding Spotlight Mode 4SPACEBORNE/AIRBORNE(星载/机载),BISTATIC5Spaceborne/Airborne Hybrid Bistatic SAR,Wavenumber-Domain(波数域) Algorithm6Sliding Spotlight and TOPS SAR,Baseband Azimuth Scaling(基带方位尺度)7INVERSE SLIDING SPOTLIGHT IMAGING8KEY PARAMETERS IN SLIDING SPOTLIGHT SAR9A STUDY OF SAR SIGNAL ANALYSIS,SLIDING SPOTLIGHT MODE 10Azimuth Ambiguity of Phased Array11Anti-Jamming(抗干扰)Property12USING EXTENDED FREQUENCY(扩展频率)SCALING13MULTIPLE SAR MODES WITH BASEBAND AZIMUTH SCALING 14With PAMIR and TerraSAR-X—Setup, Processing, and Image Result15Two-Step Algorithm in Sliding Spotlight Space-borne16 Frequency-Domain,for Spaceborne/AirborneConfiguration17 KOMPSAT-5 SPOTLIGHT SAR PROCESSOR, USING FSA WITH CALCULATION OF EFFECTIVE VELOCITY18 Time-Frequency,High-Resolution19 A Special Point Target Reference Spectrum20 Hybrid(混合式) Bistatic SAR TerraPAMIR,Geometric Description and Point Target Simulation(几何描述与点目标仿真)21Using Azimuth Frequency De-ramping(方位频率去斜)22Sliding Spotlight,TOPS SAR Data,Without Subaperture (子孔径)23 EXTENDED THREE-STEP FOCUSING ALGORITHM24The Study of realization method(实现方法)25Double Sliding Spotlight Mode with TerraSAR-X and PAMIR Based on Azimuth Chirp Filtering26A Unified(统一的) Focusing Algorithm(UFA),Based on FrFT(fractional(分数) Fourier transform)27 A MULTI-MODE SPACE-BORNE,BASED ON SBRAS(Space-borne Radar Advance Simulator)(星载雷达超前模拟器)28PRESENCE OF SQUINT(下斜视)29Large-Scene,Multiple Channels in Azimuth30Full-Aperture Azimuth,for Beam Steering (光束控制)SAR31Beam Steering (光束控制)SAR Data Processing by a Generalized PFA32Multichannel,Ultrahigh-Resolution(超高分辨率) and Wide-Swath Imaging(宽测绘带成像)33A Multi-mode Space-borne SAR34Processing of Ultrahigh-Resolution Space-borne Sliding Spotlight SAR Data on Curved Orbit(曲线轨迹)35Multichannel Sliding Spotlight and TOPS Synthetic Aperture Radar Data36Burst Mode Synthetic Aperture Radar(突发模式合成孔径雷达)37Novel High-Order Range Model(新的高阶模型),Imaging Approach for High-Resolution LEO(低轨) SAR38FULL-APERTURE IMAGING ALGORITHM39Azimuth Resampling Processing for Highly Squinted (大斜视)Synthetic Aperture Radar Imaging With Several Modes40Full-Aperture SAR,Squinted Sliding-Spotlight Mode 41X-Band SAR,TerraSAR-X,Next Generation and World SAR Constellation(一系列)42Multichannel Full-aperture,Beam Steering SAR43MONITORING THE DEFORMATION(变形监测) OF SHUPING LANDSLIDE(树坪滑坡)44USING A RANDOMLY STEERED SPOTLIGHT(随机转向聚焦)45THREE-STEP FOCUSING ALGORITHM(三步聚焦算法)ON SPATIAL VARIATION CHARACTERISTIC(空间变化特征)46 ATTITUDE STEERING STRATEGY(态度转向战略),AGILE SMALL SAR SATELLITE(敏捷小卫星)47A REFINED GEOMETRIC(几何) CORRECTION ALGORITHM FOR SPOTLIGHT AND SLIDING48EFFECTS OF PRF VARIATION ON SPACEBORNE SAR IMAGING 49Image Formation Processing,With Stepped Frequency Chirps50Fast processing of very high resolution and/or very long range airborne SAR images.51TerraSAR-X Staring52Imaging for MIMO(Multiple-input/output) Sliding Spotlight53An Azimuth Resampling,Highly Squinted Sliding Spotlight and TOPS SAR54Beam Steering SAR Data Processing By a Generalized PFA(polar formation algorithm)极坐标格式算法55 computational efficient high resolution algorithm56 An Efficient Approach With Scaling Factors(变标因子) for TOPS-Mode SAR Data FocusingTOPS1TOPS-Mode Raw Data Processing with CSA2New DOA(波达方向) Estimator for Wideband Signals3Extended Chirp Scaling4Processing of Sliding Spotlight and TOPS SAR Data Using Baseband Azimuth Scaling5TerraSAR-X,Mode Design and Performance Analysis6Multichannel Azimuth Processing,ScanSAR(扫描式雷达)and TOPS7Resolution Improvement of Wideband DOA Estimation “Squared-TOPS”(方顶)8INVESTIGATIONS ON TOPS INTERFEROMETRY(干涉测量法) WITH TERRASAR-X9Efficient Full Aperture Processing10TOPS Interferometry(干涉测量法)with TerraSAR-X.11TOPS Sentinel-1 and TerraSAR-X Processor Comparison仿真数据12An Efficient Approach With Scaling Factors13Sliding Spotlight and TOPS SAR Data Processing Without Subaperture(子孔径)14Using the Moving Band Chirp Z-Transform15EXTENDED THREE-STEP FOCUSING ALGORITHM16Scalloping(扇形) Correction in TOPS Imaging Mode SAR Data17 重复18TOPS Mode Raw Data Generation From Wide-Beam SAR Imaging Modes19An Azimuth Frequency Non-Linear Chirp Scaling(FNCS) Algorithm for TOPS SAR Imaging With High Squint Angle 20Using Chirp Scaling Algorithm21Multichannel Sliding Spotlight and TOPS Synthetic Aperture Radar Data22A COMBINED MODE OF TOPS AND INVERSE TOPS FOR MECHANICAL BEAM STEERING(机械波束转向) SPACE-BORNE SAR 组合模式23on Full-Aperture Multichannel Azimuth Data Processing 24OPERATIONAL STACKING(操作层)OF TERRASAR-X SCANSAR(扫描雷达) AND TOPS DATA25SIGNAL PROPERTIES(信号特性) OF TOPS-BASED NEAR SPACE SLOW-SPEED SAR26DOPPLER-RELATED FOCUSING ASPECTS27Squinted TOPS SAR Imaging Based on Modified Range Migration Algorithm and Spectral Analysis(改进范围迁移算法及频谱分析)28Doppler-Related Distortions in TOPS SAR Images(多普勒相关的扭曲)29A Subaperture Imaging Algorithm to Highly Squinted TOPS SAR Based on SPECAN and Deramping(处理与去斜)30An Azimuth Resampling based Imaging Algorithm for Highly Squinted Sliding Spotlight and TOPS SAR三、●MOTION COMPENSATION●Modification of SAR Step Transform●Precision SAR Processing Using Chirp Scaling●Highly Squinted Data Using a Chirp Scaling Approach withIntegrated Motion Compensation●Strip-Map(条形图)SAR Autofocus●HYBRID(混合)STRIP-MAP(带状地形图)/SPOTLlGHT SAR●Polarimetric SAR(极化SAR) for a Comprehensive TerrainScene(地形场景) Using the Mapping and Projection Algorithm (用映射和投影的方法)9717 SIFFT SAR Processing Algorithm6982 Using Noninteger(非整数) Nyquist SVA(空间变迹) Technique3232PFA(极性坐标形式算法) algorithm●the Compensation of the SAR Range Cell Migration Basedon the Chirp Z-Transform●Chirp Scaling Approach,for Processing Squint Mode●HIGH RESOLUTION,USING RANDOM PULSE TIMING(随机脉冲定时)●Extended Chirp Scaling Algorithm(ECSA),Stripmap andScanSAR Imaging Modes●Motion compensation using SAR autofocus●Signal Properties of Spaceborne Squint-Mode SAR●the Extended Chirp Scaling(ECSA)●High Quality Spotlight SAR Processing AlgorithmDesigned for LightSAR Mission●rate allocation (速度分配) for Spotlight SAR Phase HistoryData Compression●An Extension to Range-Doppler SAR Processing to AccommodateSevere Range Curvature(适应严重的距离弯曲)●Frequency Scaling Algorithm(FSA)● Time-Varying Step-Transform Algorithm for High Squint SARImaging●Without azimuth oversampling in range migration algorithm ●High-speed focusing algorithm for circular syntheticaperture radar (C-SAR)●22 Two-step Spotlight SAR Data Focusing Approach●Motion Compensation●New Applications of Nonlinear Chirp Scaling●New Subaperture Approach,High Squint SAR● a Two-Step Processing Approach●Sub-aperture algorithm fo r motion compensation improvementin wide-beam SAR data processing●Multibaseline(多基线) ATI-SAR(Abstract-Advanced,along-track,interferometry干涉测量法)for Robust Ocean Surface Velocity Estimation in Presence of Bimodal(双峰的) Doppler Spectrum●FOPEN SAR Imaging Using UWB(超宽带) Step-Frequency(步进频率) and Random Noise Waveforms能够穿透叶簇并发现隐蔽于叶簇的目标,具有极其重要的军事作用。
量子纠缠 双缝干涉 英语 范例

量子纠缠双缝干涉英语范例Engaging with the perplexing world of quantum entanglement and the double-slit interference phenomenon in the realm of English provides a fascinating journey into the depths of physics and language. Let's embark on this exploration, delving into these intricate concepts without the crutchesof conventional transition words.Quantum entanglement, a phenomenon Albert Einstein famously referred to as "spooky action at a distance," challengesour conventional understanding of reality. At its core, it entails the entwining of particles in such a way that the state of one particle instantaneously influences the stateof another, regardless of the distance separating them.This peculiar connection, seemingly defying the constraints of space and time, forms the bedrock of quantum mechanics.Moving onto the enigmatic realm of double-slit interference, we encounter another perplexing aspect of quantum physics. Imagine a scenario where particles, such as photons or electrons, are fired one by one towards a barrier with twonarrow slits. Classical intuition would suggest that each particle would pass through one of the slits and create a pattern on the screen behind the barrier corresponding tothe two slits. However, the reality is far more bewildering.When observed, particles behave as discrete entities, creating a pattern on the screen that aligns with the positions of the slits. However, when left unobserved, they exhibit wave-like behavior, producing an interferencepattern consistent with waves passing through both slits simultaneously. This duality of particle and wave behavior perplexed physicists for decades and remains a cornerstoneof quantum mechanics.Now, let's intertwine these concepts with the intricate fabric of the English language. Just as particles become entangled in the quantum realm, words and phrases entwineto convey meaning and evoke understanding. The delicate dance of syntax and semantics mirrors the interconnectedness observed in quantum systems.Consider the act of communication itself. When wearticulate thoughts and ideas, we send linguistic particles into the ether, where they interact with the minds of others, shaping perceptions and influencing understanding. In this linguistic entanglement, the state of one mind can indeed influence the state of another, echoing the eerie connectivity of entangled particles.Furthermore, language, like quantum particles, exhibits a duality of behavior. It can serve as a discrete tool for conveying specific information, much like particles behaving as individual entities when observed. Yet, it also possesses a wave-like quality, capable of conveying nuanced emotions, cultural nuances, and abstract concepts that transcend mere words on a page.Consider the phrase "I love you." In its discrete form, it conveys a specific sentiment, a declaration of affection towards another individual. However, its wave-like nature allows it to resonate with profound emotional depth, evoking a myriad of feelings and memories unique to each recipient.In a similar vein, the act of reading mirrors the double-slit experiment in its ability to collapse linguistic wave functions into discrete meanings. When we read a text, we observe its words and phrases, collapsing the wave of potential interpretations into a singular understanding based on our individual perceptions and experiences.Yet, just as the act of observation alters the behavior of quantum particles, our interpretation of language is inherently subjective, influenced by our cultural background, personal biases, and cognitive predispositions. Thus, the same text can elicit vastly different interpretations from different readers, much like the varied outcomes observed in the double-slit experiment.In conclusion, the parallels between quantum entanglement, double-slit interference, and the intricacies of the English language highlight the profound interconnectedness of the physical and linguistic worlds. Just as physicists grapple with the mysteries of the quantum realm, linguists navigate the complexities of communication, both realmsoffering endless opportunities for exploration and discovery.。
无人深空中英文对照
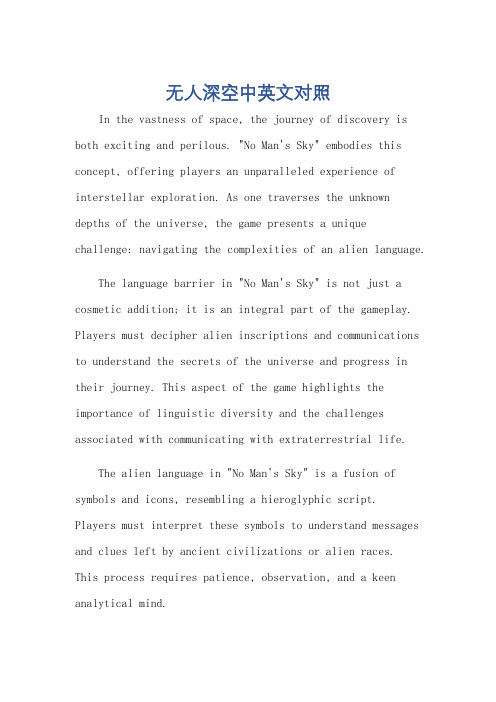
无人深空中英文对照In the vastness of space, the journey of discovery is both exciting and perilous. "No Man's Sky" embodies this concept, offering players an unparalleled experience of interstellar exploration. As one traverses the unknown depths of the universe, the game presents a unique challenge: navigating the complexities of an alien language. The language barrier in "No Man's Sky" is not just a cosmetic addition; it is an integral part of the gameplay. Players must decipher alien inscriptions and communications to understand the secrets of the universe and progress in their journey. This aspect of the game highlights the importance of linguistic diversity and the challenges associated with communicating with extraterrestrial life.The alien language in "No Man's Sky" is a fusion of symbols and icons, resembling a hieroglyphic script.Players must interpret these symbols to understand messages and clues left by ancient civilizations or alien races.This process requires patience, observation, and a keen analytical mind.The game's developers have gone to great lengths to create a believable and immersive alien language. The symbols and icons are designed to be both visually appealing and meaningful, reflecting the culture and technology of the alien races. This attention to detail adds authenticity to the game's universe and enhances the player's immersion in the experience.As players progress through the game, they encounter a diverse array of alien species, each with their own unique language and culture. This aspect of the game promotes cultural diversity and understanding, encouraging players to embrace the differences they encounter in their interstellar journey.The challenge of deciphering alien language is not just a test of the player's intellectual capabilities; it is also a test of their perseverance and dedication. The process can be frustrating at times, but the sense of achievement and satisfaction when a message is finally understood is immense. This aspect of the game fosters a sense of accomplishment and accomplishment in players,encouraging them to persevere in their exploration of the universe.In addition to the alien language, "No Man's Sky" also features a robust trading system that allows players to buy and sell goods and services with alien races. This aspectof the game adds an economic dimension to the interstellar exploration, requiring players to manage their resourcesand make strategic decisions about their trading activities. The trading system in the game is designed to be both challenging and rewarding. Players must negotiate withalien races, taking into account their different values, cultures, and trading preferences. Successful trading requires a balance of diplomacy, strategy, and market knowledge.The economic aspects of "No Man's Sky" also extend tothe player's spacecraft and equipment. Players can upgrade their ships and gear by purchasing new components and technology from alien traders. This aspect of the game encourages players to invest in their exploration capabilities, allowing them to explore deeper into the universe and discover new secrets.The graphics and sound design of "No Man's Sky" arealso noteworthy. The game's vast and detailed universe is brought to life with stunning visuals and immersive soundscapes. The alien races and environments are designedto be both visually appealing and believable, creating a truly immersive experience for players.The music of the game complements the visuals perfectly, adding emotional depth to the interstellar journey. The soundtrack features a mix of ambient and orchestral music, creating a sense of awe and wonder as players traverse the unknown depths of space.In conclusion, "No Man's Sky" is an exceptional gamethat offers players an unparalleled experience ofinterstellar exploration. The game's focus on alien language and trading systems adds a unique layer of complexity and depth to the gameplay, fostering a sense of accomplishment and engagement in players. The graphics, sound design, and immersive universe create a truly captivating experience that is sure to delight space exploration enthusiasts and casual gamers alike.**无人深空:星际探索的未知之旅**在浩瀚无垠的宇宙中,探索的旅程既令人兴奋又充满危险。
光谱层英文版

光谱层英文版The Spectral Layer: Unveiling the Invisible RealmThe universe we inhabit is a tapestry of intricately woven elements, each thread contributing to the grand tapestry of existence. Amidst this intricate web, lies a realm that is often overlooked, yet holds the key to unlocking the mysteries of our reality. This realm is the spectral layer – a realm that transcends the boundaries of our visible world and delves into the unseen realms of energy and vibration.At the heart of the spectral layer lies the electromagnetic spectrum –a vast and diverse range of wavelengths and frequencies that encompass the entirety of our physical world. From the low-frequency radio waves to the high-energy gamma rays, the electromagnetic spectrum is the foundation upon which our understanding of the universe is built. It is within this spectrum that we find the familiar visible light, the spectrum of colors that we perceive with our eyes, but it is only a small fraction of the vast and diverse tapestry that makes up the spectral layer.Beyond the visible spectrum, there lies a realm of unseen energies that are integral to the very fabric of our existence. Infrared radiation, for instance, is a form of electromagnetic radiation that is invisible to the human eye but plays a crucial role in the transfer of heat and the functioning of various biological processes. Similarly, ultraviolet radiation, though invisible to us, is essential for the production of vitamin D and the regulation of circadian rhythms.But the spectral layer extends far beyond the confines of the electromagnetic spectrum. It is a realm that encompasses the vibrations and frequencies of all matter and energy, from the subatomic particles that make up the building blocks of our universe to the vast cosmic structures that span the vastness of space. These vibrations and frequencies, though often imperceptible to our senses, are the foundation upon which the entire universe is built.At the quantum level, the spectral layer reveals the true nature of reality. Subatomic particles, such as electrons and quarks, are not merely static entities but rather dynamic oscillations of energy, each with its own unique frequency and vibration. These vibrations, in turn, give rise to the fundamental forces that govern the behavior of matter and energy, from the strong nuclear force that holds the nucleus of an atom together to the mysterious dark energy that drives the expansion of the universe.But the spectral layer is not merely a realm of the infinitely small. It also encompasses the vast and expansive structures of the cosmos, from the intricate patterns of galaxies to the pulsing rhythms of celestial bodies. The stars that dot the night sky, for instance, are not merely points of light but rather vast nuclear furnaces, each emitting a unique spectrum of electromagnetic radiation that can be detected and analyzed by scientists.Through the study of the spectral layer, we have gained unprecedented insights into the nature of our universe. By analyzing the spectra of distant galaxies, for example, we can determine their chemical composition, their age, and even their rate of expansion –information that is crucial for our understanding of the origins and evolution of the cosmos.But the spectral layer is not just a realm of scientific inquiry – it is also a realm of profound spiritual and metaphysical exploration. Many ancient and indigenous cultures have long recognized the importance of the unseen realms of energy and vibration, and have developed sophisticated systems of understanding and interacting with these realms.In the traditions of Hinduism and Buddhism, for instance, the concept of the chakras – the seven energy centers that are believed to govern various aspects of our physical, emotional, and spiritualwell-being – is a manifestation of the spectral layer. These energy centers are believed to be connected to specific frequencies and vibrations, and the practice of chakra meditation and balancing is seen as a way to align oneself with the natural rhythms of the universe.Similarly, in the traditions of shamanism and indigenous healing practices, the concept of the "spirit world" or the "unseen realm" is closely tied to the spectral layer. Shamans and healers are often said to be able to perceive and interact with the unseen energies that permeate our world, using techniques such as drumming, chanting, and plant medicine to access these realms and bring about healing and transformation.In the modern era, the spectral layer has become the subject of intense scientific and technological exploration. From the development of advanced imaging technologies that can reveal the unseen structures of the human body to the creation of sophisticated communication systems that harness the power of the electromagnetic spectrum, the spectral layer has become an essential component of our understanding and manipulation of the physical world.Yet, despite the immense progress we have made in our understanding of the spectral layer, there is still much that remainsunknown and mysterious. The nature of dark matter and dark energy, for instance, remains one of the greatest unsolved puzzles in modern physics, and the true nature of consciousness and the relationship between the physical and the metaphysical realms continues to be a subject of intense debate and exploration.As we continue to delve deeper into the spectral layer, we may uncover even more profound insights into the nature of our reality. Perhaps we will discover new forms of energy and vibration that have yet to be detected, or perhaps we will find that the boundaries between the seen and the unseen are far more permeable than we ever imagined. Whatever the future may hold, one thing is certain: the spectral layer will continue to be a source of fascination, inspiration, and mystery for generations to come.。
The properties of quantum dots
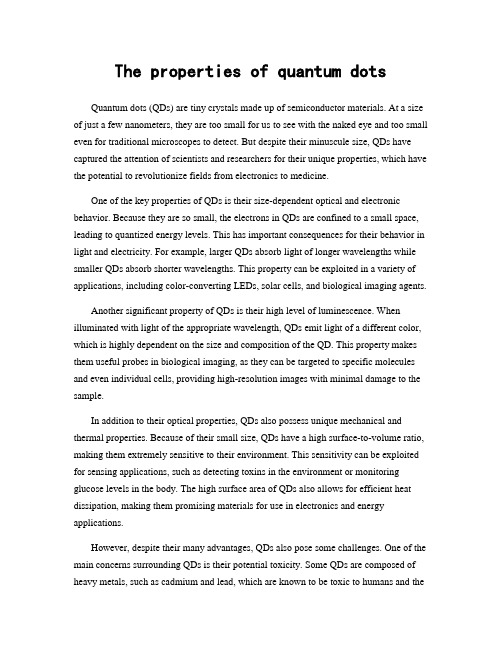
The properties of quantum dotsQuantum dots (QDs) are tiny crystals made up of semiconductor materials. At a size of just a few nanometers, they are too small for us to see with the naked eye and too small even for traditional microscopes to detect. But despite their minuscule size, QDs have captured the attention of scientists and researchers for their unique properties, which have the potential to revolutionize fields from electronics to medicine.One of the key properties of QDs is their size-dependent optical and electronic behavior. Because they are so small, the electrons in QDs are confined to a small space, leading to quantized energy levels. This has important consequences for their behavior in light and electricity. For example, larger QDs absorb light of longer wavelengths while smaller QDs absorb shorter wavelengths. This property can be exploited in a variety of applications, including color-converting LEDs, solar cells, and biological imaging agents.Another significant property of QDs is their high level of luminescence. When illuminated with light of the appropriate wavelength, QDs emit light of a different color, which is highly dependent on the size and composition of the QD. This property makes them useful probes in biological imaging, as they can be targeted to specific molecules and even individual cells, providing high-resolution images with minimal damage to the sample.In addition to their optical properties, QDs also possess unique mechanical and thermal properties. Because of their small size, QDs have a high surface-to-volume ratio, making them extremely sensitive to their environment. This sensitivity can be exploited for sensing applications, such as detecting toxins in the environment or monitoring glucose levels in the body. The high surface area of QDs also allows for efficient heat dissipation, making them promising materials for use in electronics and energy applications.However, despite their many advantages, QDs also pose some challenges. One of the main concerns surrounding QDs is their potential toxicity. Some QDs are composed of heavy metals, such as cadmium and lead, which are known to be toxic to humans and theenvironment. Researchers are working to develop safer QDs made from non-toxic materials, such as zinc and selenium, and to develop methods for removing toxic QDs from the environment.Another challenge facing QD research is their production and scalability. Currently, QDs are produced in small batches using complex chemical synthesis methods, making them expensive and time-consuming to produce. However, researchers are exploring new methods for producing QDs, such as biologically-inspired synthesis using enzymes and bacteria, which could make QDs more accessible for a variety of applications.In conclusion, QDs possess many unique and useful properties, from their size-dependent optical behavior to their high level of luminescence and thermal properties. Though they present some challenges, including toxicity and scalability, ongoing research is exploring new methods and materials to overcome these obstacles and unlock the full potential of QDs for a wide range of applications. As our understanding of these tiny crystals continues to evolve, QDs may prove to be a valuable tool for advancing fields from electronics to medicine and beyond.。
为什么望远镜能看到太空英语作文
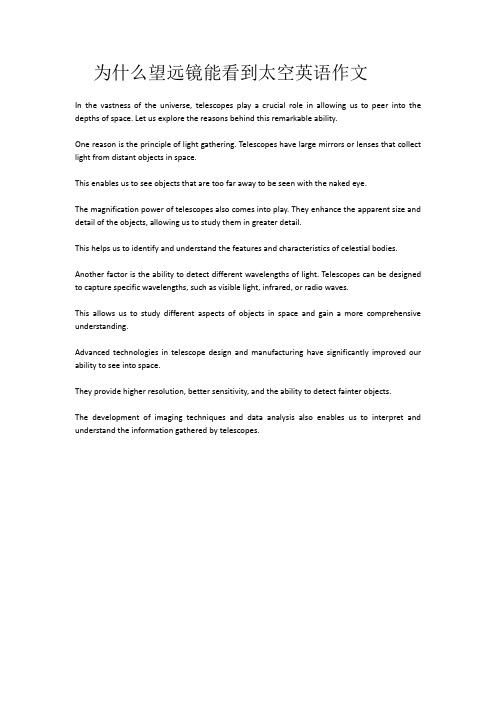
为什么望远镜能看到太空英语作文In the vastness of the universe, telescopes play a crucial role in allowing us to peer into the depths of space. Let us explore the reasons behind this remarkable ability.One reason is the principle of light gathering. Telescopes have large mirrors or lenses that collect light from distant objects in space.This enables us to see objects that are too far away to be seen with the naked eye.The magnification power of telescopes also comes into play. They enhance the apparent size and detail of the objects, allowing us to study them in greater detail.This helps us to identify and understand the features and characteristics of celestial bodies.Another factor is the ability to detect different wavelengths of light. Telescopes can be designed to capture specific wavelengths, such as visible light, infrared, or radio waves.This allows us to study different aspects of objects in space and gain a more comprehensive understanding.Advanced technologies in telescope design and manufacturing have significantly improved our ability to see into space.They provide higher resolution, better sensitivity, and the ability to detect fainter objects.The development of imaging techniques and data analysis also enables us to interpret and understand the information gathered by telescopes.。
- 1、下载文档前请自行甄别文档内容的完整性,平台不提供额外的编辑、内容补充、找答案等附加服务。
- 2、"仅部分预览"的文档,不可在线预览部分如存在完整性等问题,可反馈申请退款(可完整预览的文档不适用该条件!)。
- 3、如文档侵犯您的权益,请联系客服反馈,我们会尽快为您处理(人工客服工作时间:9:00-18:30)。
Signal Properties of Spaceborne Squint-Mode SAR Gordon W.Davidson,Member,IEEE,and Ian Cumming,Member,IEEEAbstract—This paper presents signal properties of spaceborne, strip-map SAR for very large squint angles.Doppler centroid, azimuth bandwidth,and exposure time are derived in terms of the platform attitude and elevation angle.This allows a description of the Doppler centroid variation with range and terrain height,and explains a possible range dependence of azimuth bandwidth at high squint.The yaw and pitch required to minimize Doppler centroid and azimuth bandwidth variation are derived,and residual Doppler centroid variation in the presence of antenna pointing errors is presented.The SAR constraint on the relationship between swath width and azimuth bandwidth is revisited for high squint,and is used to present a fundamental limit on squint angle.Finally,processing considerations arising from the shape of the two-dimensional(2-D)spectrum with high squint are discussed.I.I NTRODUCTIONS IGNAL properties of spaceborne,strip-map synthetic aperture radar(SAR),such as Doppler centroid and azimuth bandwidth,are well understood for the conventional case in which the antenna is pointed nearly perpendicular to theflight path.In squint mode,the antenna is pointed forward or backward from the perpendicular position by a squint angle that is as much as several tens of degrees.Squint mode has been used in airborne platforms,and for this case Doppler properties are typically described by the orientation of the antenna footprint compared to iso-Doppler lines on aflat surface[1].However,signal properties are difficult to quantify with this approach in the case of a general earth geometry as with a spaceborne platform and in the case that terrain height variation is to be taken into account.In this paper we present a general approach to describ-ing high squint SAR signal properties,without small angle approximations,in terms of the platform attitude and eleva-tion angle.This is valid for any orbit and earth geometry, including terrain height,and the effects on signal properties of antenna pointing errors from a desired attitude are readily obtained.In particular,the variation of Doppler centroid with range and terrain height,and a possible range-dependence of azimuth bandwidth,are investigated.Range variation of the Doppler centroid can lead to different wraparound of azimuth frequencies at different ranges,after sampling by the pulse repetition frequency(PRF).This can be accommodated by replication of azimuth spectra[2],but if the variation is large Manuscript received June29,1995;revised August22,1996.This work was supported by the B.C.Science Council,the Natural Science and Engineering Research Council of Canada,the B.C.Advanced Systems Institute,and MacDonald Dettwiler and Associates.G.W.Davidson is with the Advanced Development Group,Glenayre, Vancouver,B.C.,Canada(e-mail:gdavidso@).I.Cumming is with the Department of Electrical Engineering,University of British Columbia,Vancouver,B.C.,Canada V6T1Z4.Publisher Item Identifier S0196-2892(97)00378-1.it can significantly increase the computation of processing. Doppler centroid variation with terrain height can lead to undersampling and azimuth ambiguities.Finally,the behavior of azimuth bandwidth is important in terms of maintaining a PRF that satisfies the SAR constraint offitting a received echo between transmitted pulses.It is shown how,with proper attitude steering,Doppler centroid variation is minimized,and typical SAR properties are preserved in squint mode,allowing data to be collected accurately and efficiently processed in the two-dimensional(2-D)frequency domain.The high squint results are general and therefore provide a thorough understanding of the SAR signal.Furthermore, squint mode has the potential to provide information such as the azimuth angle dependence of backscatter.Since the visibility of structural features on the surface can depend on the viewing angle,this would aid the interpretation of SAR imagery in complex terrain[3].Other uses of squint can be envisioned,such asfilling in shadow areas,or illuminating adjacent range subswaths at different times as the platform passes the desired area:in general,increasing theflexibility with which a particular area on the surface can be imaged within a single pass of the platform.Also,the application of squint is suited to a spaceborne platform,where theflight direction over the surface is determined by the orbit and cannot be changed significantly.Section II describes the general SAR geometry,the notation, and thefirst part of equations describing signal properties at high squint.Section III completes the properties by describing squint and squinted beamwidth in terms of yaw,pitch,and elevation angle.Also,the yaw and pitch required to minimize Doppler centroid variation and maintain a range-independent azimuth bandwidth are derived,and residual Doppler centroid variation with antenna pointing errors are presented.Section IV discusses the SAR constraint offitting the received echo between transmitted pulses in the context of high squint,and a fundamental limit on squint is shown.Section V discusses the2-D spectrum of the SAR signal at high squint and considerations for processing.Finally,Section VI gives the conclusions.II.G ENERAL P ROPERTIESFig.1illustrates the general spaceborne SAR geometry for a high squint angle.The platform position at azimuthtime,.The velocity is assumed to be relative to the scatterer,so it includes the effect of earth rotation,and the plane perpendicularto,and the closest approachrange,,and0196–2892/97$10.00©1997IEEEFig.1.Squint mode SAR imaging geometry.togetherwith.The magnitudeof ,which is the azimuth-varying distance from the platform to thescatterer,,is known from the orbital data and earth model [4].It is useful to define an instantaneous squint angle to thescatterer,,which is measured within the slant range plane,from the zero Doppler planeto .The instanta-neous squint angle varies with azimuth,and is equal to the antenna boresight squintangle,,and the squinted beamwidth are basicparameters that determine signal properties,and will be related to platform attitude and elevation angle in the next section.In the SAR signal received from a point scatterer,the varying distance to a scatterer,with respect to the wavelength,causes an azimuth-varying phase in the signal.This results in an instantaneous azimuth (Doppler)frequency,(1)where is the wavelength.The center of signal energy in the azimuth fre-quency domain is the Doppler centroid,which is the azimuth frequency when the scatterer is at the center of thebeam.Finally,the elevation angle is measured in a plane perpen-dicular to the orbital plane.For the low squint case the usual elevation angle is measured at the scatterer’s azimuthposition,,and is indicatedbyby,closest approachrange,,and terrainheight,(7)whereis the nominal earth radius,and-axis is in the direction of the velocity vector of the platform relative to the earth,the-axis completes the orthogonalDA VIDSON AND CUMMING:SIGNAL PROPERTIES OF SPACEBORNE SQUINT-MODE SAR613Fig.2.Definition of yaw and pitch rotations from zero-Doppler coordinate system.set.For purposes of describing the squint angle,only yaw and pitch rotations are described,since the results are to be described for any elevation angle.The first illustration shows an initial zero-Doppler vector at an initial elevationangle,,is assumed to be done first and isa rotation aboutthe,is a rotation abouttheyaw-rotated,and a final elevationangle,,and for narrow beamwidths thisisFig.3.Squinted beamwidth.Illustration of elevation angles at beam edges before and after squinting.approximately(13)To find an expression for squinted beamwidth,start with initial vectors that point to the leading and trailing azimuth edges of the zero squint footprint and apply the yaw and pitch rotations to get the squinted vectors at the leading and trailing edges of the squinted footprint.The instantaneous squint angles of the initial vectors,before rotation,areapproximatelyis the azimuth antenna length.Afterrotation,the vectors should be aligned in such a way that a scatterer passes through the squinted footprint at a constant elevationangle,and ,from (10).However,calculations with typical SAR parameters showed that the changeinand ,as shownin Fig.3.Applying the yaw and pitch rotation to these initial vectors,and using the relationship between initial and final elevation angles,gives”)and trailing (“notation.Also obtained from the rotations of these initial vectors are expressionsforand .Substituting into (13)and rearranging gives the expression for squintedbeamwidth(15)Equations (9)and (15)give the squint and squinted beamwidth,and hence the Doppler centroid,azimuth bandwidth,and exposure time,for a scatterer at the given elevation.In particular,the general expression for squinted beamwidth is seen to depend on squint and elevation angle,which is not normally assumed in SAR,and the effect on azimuth bandwidth will be seen in the next subsection.614IEEE TRANSACTIONS ON GEOSCIENCE AND REMOTE SENSING,VOL.35,NO.3,MAY1997B.Attitude ControlThe dependence of squint on elevation means that thesquint angle,and hence Doppler centroid,will vary acrossthe elevation beamwidth,resulting in the variation of Dopplercentroid with range and terrain height.It is known that correctchoice of yaw and pitch can minimize the variation of Dopplercentroid with range,by aligning the footprint with an iso-Doppler line[7].Here,we present the solution for yawand pitch to minimize the variation of squint with elevation,without small angle approximations,thus minimizing Dopplercentroid variation with range and terrain height for any earthgeometry.The solution for the yaw and pitch is evaluated ata nominal elevation angle corresponding to midswath and anaverage terrain height.The residual Doppler centroid variation,including the effect of errors in yaw and pitch,is thenpresented.The rate of change of squint with elevation is minimizedbysettingis nonzero andfinite,it can be shown that to minimize squintvariation with elevation it is sufficient tosatisfy(18)Thus,given a desired squintangle,,(9),(10),and(11)can be written interms of the three unknowns:yaw,pitch,and a nominal initialelevation angle.These equations can be solved for therequired(19)(20),andand(22)Tofind the residual Doppler centroid variation for varioussquint angles,the optimum yaw and pitch angles were calcu-lated for an elevation angle at midswath and nominal terrainheight,and antenna pointing errors were added.Then,theelevation angles were calculated at the edges of theswath,Fig.4.Doppler centroid error versus squint,due to range variation and1000m height variation,for Seasat(L-band)and ERS-1(C-band)parameters.Solidcurve indicates0 antenna pointing error,dashed curve indicates60.5 error,and dotted curve indicates61 error.and with a terrain height changeof m.This wasused tofind the difference in squint angle from the desiredsquint angle,which in turn was used tofind the residualDoppler centroid error.The parameters of the calculationswere chosen to be representative of the platforms,Seasat,ERS-1,and X-SAR,with wavelengths at L-band,C-band,andX-band,respectively.For the satellite platforms,Seasat andERS-1,the altitude is800km and the ground swath widthis100km,and the nominal elevation angle at midswath is21,respectively.Figs.4and5show the residual Doppler centroid error,inHertz,versus squint angle in degrees for the different cases.Results are given for different values of maximum antennapointing error:00.51PBW),where PBW is the processed azimuth bandwidth,sothat the Doppler centroid variation should typically be lessthan about200to300Hz for spaceborne platforms.It ispossible to accommodate some range variation of Dopplercentroid during processing in the two-dimensional frequencydomain,but a significant variation of Doppler centroid withterrain height will lead to azimuth ambiguities that cannot beeasily accommodated in processing.As can be seen in Figs.4and5,the proper choice ofyaw and pitch angles allows the collection of SAR datawith acceptable Doppler centroid variation in most cases.Antenna pointing errors increase the residual Doppler centroidvariation,although the variation is almost as large at nominallyzero squint as it is at higher squint angles.The residual Dopplercentroid variation actually decreases with squint angles pastDA VIDSON AND CUMMING:SIGNAL PROPERTIES OF SPACEBORNE SQUINT-MODE SAR615Fig.5.Doppler centroid error versus squint,due to range variation and 1000m height variation,for X-SAR (X-band)parameters at near incidence (21 )and far incidence (45 ).Solid curve indicates 0 antenna pointing error,dashed curve indicates 60.5 error,and dotted curve indicates 61 error.about 30antennapointing error.At C-band (ERS-1)the range variation is barely acceptable with a 0.5pointing error.The terrain height variation of Doppler centroid with ERS-1parameters is acceptable.With the small X-band wavelength,Doppler centroid variation is quite large,especially for the near incidence case.Also,the terrain height variation of Doppler centroid is larger for X-SAR because of the lower altitude of the space shuttle.The X-SAR,near incidence case represents the worst case considered,and the terrain height variation is possibly unacceptable in mountainous terrain.At far incidence,the Doppler centroid variation is smaller,so that the terrain height variation becomes acceptable,although the range variation would require some accommodation at a1(24)which is independent of range and decreases as the cosine of the squint angle.Thus,proper selection of yaw and pitch angles not only minimizes Doppler centroid variation,but also preserves the property of SAR imaging that the azimuth bandwidth is independent of range.Also,the dependence ofTABLE IA ZIMUTHB ANDWIDTH V ERSUS S QUINT FOR X-SAR P ARAMETERS :Y AW R OTATION O NLY ;AND WITH O PTIMAL Y AW AND PITCHazimuth bandwidthonis the speed of light,plusthe transmitted pulselength,616IEEE TRANSACTIONS ON GEOSCIENCE AND REMOTE SENSING,VOL.35,NO.3,MAY1997Fig.6.Closest approach range swath versus squint for Seasat (L-band),ERS-1(C-band),and X-SAR (X-band).echo,and a guardspace,,that allows for variations in the range delay of the signal and to allow some flexibility in choosing the PRF.This constraint can be expressedas(25)For a given closest approach rangeswath,PRF is the the value at zero squint.Also,let the guard space be proportional to the time between pulses,so(27)where the first term is the swath width at zero squint.As squint increases,the allowable swath width depends on the opposing effects represented by the last two terms in this equation.Without range migration,as the PRF decreases with increasing squint there is proportionally more room for the received echo since the transmitted pulse length stays the same.However,the increase in range migration with squint,increases the echo length and reduces the allowable swath width.This was investigated by calculating the maximum available swath width as a function of squintangle.at the closest and farthest points intheFig.7.Skewed region of support of data spectrum.swath,for spaceborne SAR geometry using parameters for Seasat,ERS-1,and X-SAR (near incidence).The maximum value of closest approach rangeswath,.For the smaller wavelength of ERS-1,the allowableswath width is larger,but still decreases noticeably with squint.For these cases it is seen how,depending on wavelength,the increased echo length due to range migration gives a fundamental limitation on squint angle.The X-SAR platform has a smaller wavelength and lower altitude,resulting in a small amount of range migration so that the allowable swath width increases slightly with squint.V.D ATA S PECTRUMAssuming precision processing in the 2-D frequency do-main,at high squint the region of support of the data spectrum poses an interesting problem,which arises from the depen-dence of the Doppler centroid on rangefrequency,DA VIDSON AND CUMMING:SIGNAL PROPERTIES OF SPACEBORNE SQUINT-MODE SAR617Fig.8.Replication of parts of spectrum to expand azimuth bandwidth. enough to alleviate this problem,because of the SAR imaging constraints discussed earlier.Thus,while the SAR data can be collected with an azimuth sampling rate that decreases with squint angle,the processing of the data into an image requires extra azimuth bandwidth to avoid aliasing.This problem occurs for any SAR processing algorithm,since the potential for aliasing is inherent in the signal.This problem can be accommodated during processing by replicating the parts of the spectrum that overlap,as illustrated in Fig.8.This produces a2-D spectrum without overlap,removing the potential for aliasing during processing. Essentially,the azimuth bandwidth is expanded to accom-modate the nature of the squinted SAR signal.Also,this replication of range lines can be done in the range-Doppler domain,before the range Fourier transform.This allows all processing steps that depend on azimuth-frequency,such as range-migration correction,to be performed correctly for all azimuth-frequencies in the signal.Then,the unwanted pieces of duplicated spectrum,shown in thefigure,can be removed in the2-D frequency domain by applying a window.VI.C ONCLUSIONGeneral properties of spaceborne,strip-map SAR which are valid for very large squint angles have been presented in terms of the squint and the squinted beamwidth,which are in turn expressed in terms of the platform attitude and elevation angle.The yaw and pitch angles which minimize the variation of Doppler centroid with range and terrain height have been derived,and calculation of the residual Doppler centroid variation in the presence of antenna pointing errors for Seasat,ERS-1,and X-SAR parameters have been shown to give acceptable results,except for the case of X-SAR at near incidence.It was shown that the correct yaw and pitch are also necessary to maintain the SAR property of an azimuth band-width that is independent of range.In this case,the azimuth bandwidth of the SAR data decreases as the cosine of the squint angle.The smaller PRF obtained with proper steering helps to satisfy the SAR constraint offitting a received echo between transmitted pulses.However,because of the increased range migration at higher squint,this SAR constraint places a fundamental limit on the squint angle for imaging a given swath width.Finally,the variation of the Doppler centroid with range frequency results in a skewed region of support of the 2-D Fourier transform of the raw data,such that parts of the repeated spectra of the sampled signal can overlap and lead to aliasing during processing.Thus,it may be necessary to replicate data at certain azimuth frequencies to avoid overlap.Although squint mode has not been previously used in spaceborne,strip-map SAR,it has been shown that with proper attitude control,such data can be collected accurately,such that typical SAR properties are preserved,and in way that allows efficient processing in the2-D frequency domain.R EFERENCES[1]R.O.Harger,Synthetic Aperture Radar Systems:Theory and Design.New York:Academic,1970.[2]I.G.Cumming,F.H.Wong,and R.K.Raney,“A SAR process-ing algorithm with no interpolation,”in IGARSS’92Proc.,1992,pp.376–379.[3]J.Way and E.A.Smith,“The evolution of synthetic aperture radarsystems and their progression to the EOS SAR,”IEEE Trans.Geosci.Remote Sensing,vol.29,pp.962–985,Nov.1991.[4] B.C.Barber,“Theory of digital imaging from orbital synthetic-apertureradar,”Int.J.Remote Sensing,vol.6,no.7,pp.1009–1057,1985. [5]R.K.Raney,“Doppler properties of radars in circular orbits,”Int.J.Remote Sensing,vol.7,no.9,pp.1153–1162,1986.[6]H.Runge,“Benefits of antenna yaw steering for SAR,”in IGARSS’91Proc.,1991,pp.257–261.[7] C.Y.Chang,M.Jin,and J.C.Curlander,“Squint mode SAR processingalgorithms,”in Proc.IGARSS’89,1989,pp.1702–1706.[8]R.Bamler and H.Runge,“PRF-ambiguity resolving by wavelengthdiversity,”IEEE Trans.Geosci.Remote Sensing,vol.29,pp.997–1003, Nov.1991.Gordon W.Davidson(S’83–M’86)received theB.Sc.in electrical engineering from the Universityof Calgary,Calgary,Alta.,Canada,in1984.Hereceived the M.Eng.degree in systems and computerengineering from Carleton University,Ottawa,Ont.,Canada,in1986,with his thesis investigating adap-tive equalization techniques on a fading channel.In1994,he received the Ph.D.degree in electricalengineering from the University of British Columbia(UBC),Vancouver, B.C.,Canada,for which heinvestigated squint mode SAR signal properties and developed high squint SAR processing based on the chirp scaling algorithm.From1986to1988,he was with Bell Northern Research,Ottawa,in software development,and in1989he was a research assistant in adaptive signal processing for communications at Carleton University.During his Ph.D. study he consulted for MacDonald Dettwiler in the area of SAR processing. In1994and1995,he was a lecturer at UBC in digital signal processing.Then from1995to1996,he was a guest scientist at the German Aerospace Research Establishment(DLR),Oberpfaffenhofen,Germany,working in ScanSAR and SAR interferometry.Since1997,he has been in the Advanced Development Group at Glenayre,Vancouver,working in wirelesscommunications.Ian Cumming(S’63–M’66)received the B.Sc.de-gree in engineering physics from the University ofToronto,Toronto,Ont.,Canada,in1961,and thePh.D.degree in computing and automation fromImperial College,University of London,London,U.K.,in1968.Following work in steel mill automation andsonar signal processing,he joined MacDonald Det-twiler and Associates in1977.Since then,he hasdeveloped synthetic aperture radar signal processingalgorithms,and has worked on systems for process-ing polarimetric and interferometric radar data,and for the compression of radar data.In1993,he joined the Department of Electrical Engineering, University of British Columbia,Vancouver,B.C.,Canada,where he holds the MacDonald Dettwiler/NSERC Industrial Research Chair in radar Remote Sensing.The associated laboratory supports a research staff of ten engineers and students working in thefields of SAR processing,SAR data encoding, satellite SAR,two-pass interferometry,airborne,along-track interferometry, airborne polarimetric radar classification,and SAR Doppler estimation.。