Biodiesel from Algae
尼罗红-荧光光谱法测定亚心形扁藻油脂含量
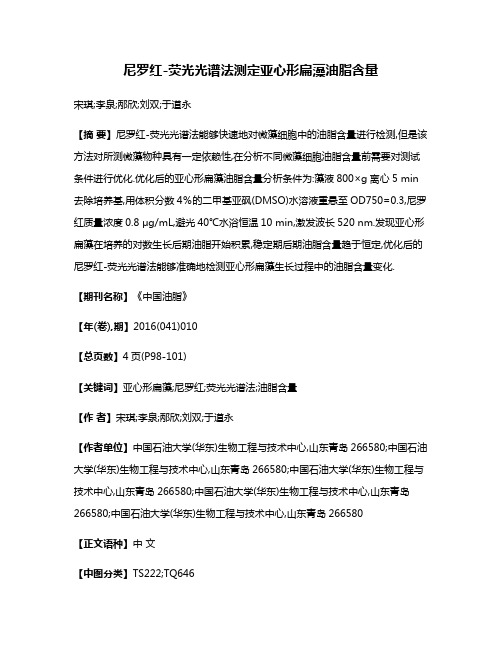
尼罗红-荧光光谱法测定亚心形扁藻油脂含量宋琪;李泉;邴欣;刘双;于道永【摘要】尼罗红-荧光光谱法能够快速地对微藻细胞中的油脂含量进行检测,但是该方法对所测微藻物种具有一定依赖性,在分析不同微藻细胞油脂含量前需要对测试条件进行优化.优化后的亚心形扁藻油脂含量分析条件为:藻液800×g离心5 min 去除培养基,用体积分数4%的二甲基亚砜(DMSO)水溶液重悬至OD750=0.3,尼罗红质量浓度0.8 μg/mL,避光40℃水浴恒温10 min,激发波长520 nm.发现亚心形扁藻在培养的对数生长后期油脂开始积累,稳定期后期油脂含量趋于恒定,优化后的尼罗红-荧光光谱法能够准确地检测亚心形扁藻生长过程中的油脂含量变化.【期刊名称】《中国油脂》【年(卷),期】2016(041)010【总页数】4页(P98-101)【关键词】亚心形扁藻;尼罗红;荧光光谱法;油脂含量【作者】宋琪;李泉;邴欣;刘双;于道永【作者单位】中国石油大学(华东)生物工程与技术中心,山东青岛266580;中国石油大学(华东)生物工程与技术中心,山东青岛266580;中国石油大学(华东)生物工程与技术中心,山东青岛266580;中国石油大学(华东)生物工程与技术中心,山东青岛266580;中国石油大学(华东)生物工程与技术中心,山东青岛266580【正文语种】中文【中图分类】TS222;TQ646随着全球能源消耗量的不断增加,不可再生的化石能源日渐短缺,而使用化石燃料还会产生大量的CO2及硫、氮氧化物等有害气体危害环境。
生物柴油是一种通过甘油三酯或游离脂肪酸与短链醇的酯基转移反应或酯化作用形成的单烷基酯,它经过稍微改性或不改性就可以在传统的柴油机中使用[1]。
生物柴油由于环境友好及可再生性,成为较理想的替代能源之一。
微藻具有光合效率高、生长周期短、含油量高等优点,是生产生物柴油的理想原料[2]。
提高微藻油脂含量是降低生物柴油成本的重要途径,是微藻生物柴油产业化亟待解决的关键问题之一。
algae DHA 藻类 多不饱和脂肪酸

Producing Docosahexaenoic Acid(DHA)-Rich Algaefrom Biodiesel-Derived Crude Glycerol:Effects ofImpurities on DHA Production and Algal BiomassCompositionD ENVER J.P YLE,†R AFAEL A.G ARCIA,§AND Z HIYOU W EN*,†,#Department of Biological Systems Engineering and Institute for Critical Technology and AppliedScience,Virginia Polytechnic Institute and State University,Blacksburg,Virginia24061,and Fats,Oils and Animal Coproducts Research Unit,Eastern Regional Research Center,Agricultural ResearchService,U.S.Department of Agriculture,600East Mermaid Lane,Wyndmoor,Pennsylvania19038Crude glycerol is the primary byproduct of the biodiesel industry.Producing docosahexaenoic acid (DHA,22:6n-3)through fermentation of the alga Schizochytrium limacinum on crude glycerol provides a unique opportunity to utilize a large quantity of this byproduct.The objective of this work is to investigate the effects of impurities contained in the crude glycerol on DHA production and algal biomass composition.Crude glycerol streams were obtained from different biodiesel refineries.All of the glycerol samples contained methanol,soaps,and various elements including calcium,phosphorus, potassium,silicon,sodium,and zinc.Both methanol and soap were found to negatively influence algal DHA production;these two impurities can be removed from culture medium by evaporation through autoclaving(for methanol)and by precipitation through pH adjustment(for soap).The glycerol-derived algal biomass contained45-50%lipid,14-20%protein,and25%carbohydrate,with8-13% ash content.Palmitic acid(C16:0)and DHA were the two major fatty acids in the algal lipid.The algal biomass was rich in lysine and cysteine,relative to many common feedstuffs.Elemental analysis by inductively coupled plasma showed that boron,calcium,copper,iron,magnesium,phosphorus, potassium,silicon,sodium,and sulfur were present in the biomass,whereas no heavy metals(such as mercury)were detected in the algal biomass.Overall,the results show that crude glycerol was a suitable carbon source for algal fermentation.The crude glycerol-derived algal biomass had a high level of DHA and a nutritional profile similar to that of commercial algal biomass,suggesting a great potential for using crude glycerol-derived algae in omega-3-fortified food or feed.KEYWORDS:Biodiesel;crude glyercol;soap;methanol;docosahexaenoic acid(DHA);algal biomass; proximate analysis;fatty acids profile;amino acid composition;elemental composition;heavy metal contaminationINTRODUCTIONIn recent years,biodiesel has become an important source of alternative fuel for the United States.The National Biodiesel Board has projected an annual production of biodiesel in2007 of450million gallons,a sharp increase from<100million gallons prior to2005(1).The major byproduct of biodiesel production is crude glycerol.In general,for every gallon of biodiesel produced,0.3kg of glycerol is generated(2).As biodiesel production skyrockets,the market is beingflooded with crude glycerol.Indeed,crude glycerol prices have dropped from$0.25/lb in2004to$0.025-0.05/lb in2006(3,4)because the current U.S.demand for glycerol is not large enough for all of this excess glycerol.It is clear that new uses for biodiesel-derived crude glycerol are needed.Several avenues for utilizing this crude glycerol have been investigated.For example,glycerol can be thermochemically converted into propylene glycol(5)or acetol(6).It can also be used in fermentation processes to produce1,3-propanediol(7), lipids(8–10),pigments(9),and a mixture of succinic acid, butanol,ethanol,and hydrogen(11).Recently,research con-ducted in our laboratory has shown that crude glycerol can be used to produce docosahexaenoic acid(DHA,22:5n-3)through fermentation of the alga Schizochytrium limacinum(12).*Address correspondence to this author at the Department ofBiological Systems Engineering,Virginia Polytechnic Institute and StateUniversity,Blacksburg,VA24061[e-mail wenz@;fax(540)231-3199].†Department of Biological Systems Engineering,Virginia Poly-technic Institute and State University.§U.S.Department of Agriculture.#Institute for Critical Technology and Applied Science,VirginiaPolytechnic Institute and State University.J.Agric.Food Chem.2008,56,3933–3939393310.1021/jf800602s CCC:$40.75 2008American Chemical SocietyPublished on Web05/09/2008DHA is an important omega-3polyunsaturated fatty acid(n-3 PUFA)that has been shown to have beneficial effects on preventing human cardiovascular diseases,cancer,schizophre-nia,and Alzheimer’s disease(13).DHA also plays an important role in infant brain and retinal development(14).In the aquaculture industry,n-3PUFAs are essential nutrients for cultured marinefish.The major commercial source of n-3 PUFAs isfish oil,which faces challenges such as odor/taste problems,heavy metal contamination,and limited supply(15). Currently,the aquaculture industry is experiencing rapid increases infish oil price due toflat supply and increased global demand for this commodity.In fact,the Food and Agriculture Organization(FAO)of the United Nations predicts thatfish oil demand in2015will be145%of historical global production capacity(16).The inability to expandfish oil production makes development offish oil alternatives imperative. Developing DHA-containing microalgae from biodiesel waste glycerol is an excellent opportunity to provide alternative omega-3sources.The fatty acids can be extracted from algae and used in fortified foods,or the biomass can be used directly as a feed additive in various animal industries such as aquac-ulture(17)or poultry(18).In addition,potential environmental impacts associated with crude glycerol disposal could be minimized.Although it has been shown that crude glycerol from the biodiesel industry can support the growth and DHA production of S.limacinum(12),a thorough chemical characterization of the algal biomass is needed before the alga-derived omega-3 fatty acids are used as food or feed additives.In general,the composition of crude glycerol varies from plant to plant;it contains methanol and soap as the two major impurities,with various elements such as calcium,potassium,phosphorus, magnesium,sulfur,and sodium present.Although the biodiesel manufacturing process does not involve any heavy metals,the oil feedstock may contain trace amounts of heavy metals,whichmay eventually end up in algal cells.The objective of this work is to investigate the effects of these impurities on algal DHA production and the“quality”of algal biomass in terms of its elemental composition and nutritional levels.Ensuring that algal biomass can be easily produced and safely utilized will open new markets for biodiesel-derived crude glycerol. MATERIALS AND METHODSAlgal Strain,Medium,and Culture Conditions.S.limacinum SR-21(ATCC MYA-1381)was used.The cells were maintained in a medium containing10g/L glucose,1.0g/L yeast extract,and1.0g/L peptone in artificial seawater.The artificial seawater contained(per liter) 18g of NaCl,2.44g of MgSO4·7H2O,0.6g of KCl,1.0g of NaNO3, 0.3g of CaCl2·2H2O,0.05g of KH2PO4,1.0g of Tris buffer(Sigma Co.),0.027g of NH4Cl,15.0×10-8g of vitamin B12,3mL of chelated iron solution,and10mL of trace element solution including boron, cobalt,manganese,zinc,and molybdenum(19).The pH was adjusted to7.5-8.0before the medium was autoclaved at121°C for15min. The cells were grown in250mL Erlenmeyerflasks,each containing 50mL of medium,and incubated at20°C in an orbital shaker set to 170rpm.Subcultured cells were used as inoculum.The inoculum size was10%of the total liquid volume in eachflask.In the study of DHA production from crude glycerol,all of the medium components were the same as those used in the subculture except that different concentrations of crude glycerol were used to replace glucose.Crude Glycerol Sources and Soap Removal Procedures.Different crude glycerol streams were tested,including(G1)glycerol derived from soybean oil by Virginia Biodiesel Refinery(West Point,VA), (G2)glycerol from a50:50(w/w)chicken fat and soybean oil mixture by Virginia Biodiesel,and(G3)glycerol from canola oil by Seattle Biodiesel LLC(Seattle,WA).Both of the biodiesel plants used alkali-catalyzed transesterification of oil with methanol to produce the biodiesel.Virginia Biodiesel used KOH as catalyst,whereas Seattle Biodiesel used NaOH as catalyst.All of the crude glycerol samples contained soaps,which were formed from a side reaction.The soaps can be split into free fatty acids (FFAs)and salt by adding a strong acid to the glycerol to neutralize the catalyst,that isDepending on the experimental conditions,soaps either remained in or were removed from the algal culture medium.To prepare soap-free medium,the following procedures were used:(i)the glycerol was mixed with distilled water at a ratio of1:4(v/v)to reduce the viscosity of thefluid,(ii)the pH of thefluid was adjusted to3with hydrochloric acid to convert soap into free fatty acids that precipitated from the liquid,(iii)precipitated free fatty acids were separated from the crude glycerol solution by centrifugation at5000rpm,and(iv)other nutrients (mineral salts,nitrogen source,etc.)were added to the glycerol solution to adjust to the desired levels.Analysis.Cell Dry Weight and Glycerol and Methanol Concentra-tions.Cell dry weight was determined as previously reported(12). Glycerol and methanol concentrations were determined by a Shimadzu Prominence HPLC System(Shimadzu Scientific Instruments,Inc., Columbia,MD).The detailed procedures were described previously (12).Proximate Analysis.Algal biomass was freeze-dried prior to proximate analysis.The lipids from the algal biomass were extracted Table1.Detectable Limits of Various Elements with ICP Analysis element detection limit(ppm)element detection limit(ppm) aluminum10nickel5 antimony20niobium50 arsenic50osmium10 barium5palladium50 berylium2phosphorus50 bismuth10platinum50boron10potassium100 cadmium2praseodymium50 calcium50rhenium50 cerium50rhodium100 chromium2ruthenium50cobalt5samarium50 copper2scandium10 dysprosium10selenium50 erbium10silicon10 europium10silver2 gadolinium20sodium50 gallium20strontium10 germanium50sulfur100gold20tantalum50 hafnium50tellurium100 holminum50terbium50 indium50thallium50iodine200thorium50 iridium50thulium50iron10tin10 lanthanum50titanium2lead20tungsten50 lithium10uranium100 lutetium10vanadium5 magnesium50ytterbium10 manganese2ytrtrium2 mercury a50zinc2 molybdenum10zirconium10 neodymium20a EPA method SW-8467471A was also used to detect trace amounts of mercury with a detection limit of0.025ppm.(1)3934J.Agric.Food Chem.,Vol.56,No.11,2008Pyle et al.and quantified according to the Bligh and Dyer method(20).The crude protein content was estimated by measuring the Kjeldahl nitrogen content and multiplying the result by6.25.The ash content was determined by heating the sample at550°C overnight and then weighing the remaining matter.The carbohydrate was then calculated by subtraction.Fatty Acid Analysis.Fatty acid compositions of the lyophilized algal biomass and of free fatty acid from the crude glycerol were determined. Fatty acid methyl esters(FAME)were prepared by direct methylation with5%methanolic HCl(21–23)and determined by a Shimadzu2010 gas chromatograph(Shimadzu Scientific Instruments)equipped with a flame ionization detector and a SGE SolGel-Wax capillary column(30 m×0.25mm×0.25µm).Helium was used carrier gas.The temperature settings for injector,column,and detector were described previously(12).The fatty acids were identified by comparing the retention times with those of standard fatty acids(Nu-Chek Prep Inc., Elysian,MN)and quantified by comparing their peak area with that of the internal standard(C17:0)(12).Amino Acid Analysis.Freeze-dried algal biomass(∼25mg)was hydrolyzed in triplicate using a PicoTag workstation(Waters Corp., Milford,MA)according to the manufacturer’s directions.Hydrolyzedsamples werefiltered,dried under vacuum,and derivatized with AccQFluor reagent(Waters)following the manufacturer’s directions. Chromatography was performed using procedures described as“mixture 1”by van Wandelen and Cohen(24),with R-aminobutyric acid as an internal standard.Separate analyses were performed for cyst(e)ine,using the method described by Finley(25)to quantitatively oxidize cysteine and cystine to cysteic acid prior to hydrolysis;these samples were then analyzed in the same manner as the other samples.Elemental Analysis.Elemental composition of the crude glycerol and algal biomass was determined by an inductively coupled plasma semi-quantitative scan of69elements according to EPA method SW-8466010B(SuperScan69performed by Prochem Analytical Inc., Elliston,VA).The elements and their detection limits are listed in Table 1.In addition,EPA method SW-8467471A was further used to detect any trace amount of mercury possibly contained in the algal biomass; the detection limit for this measurement was0.025ppm(25ppb). RESULTS AND DISCUSSIONCharacterization of Crude Glycerol.The crude glycerol used in this work had a dark brown color with a high pH level(11,12).Because the biodiesel refineries used excess methanol to drive the transesterification toward a maximum biodiesel yield,the crude glycerol contained methanol.Soap was also found in the crude glycerol due to a side reaction. Considering the difficulty to determine the exact amount of soap dissolved in the crude glycerol solution,we used free fatty acid precipitated from the crude glycerol(eq1)to roughly estimate the amount of the soap residue.As shown in Table2,glycerols G1and G2contained 12-15%methanol and23-25%soap,wheras crude glycerol from Seattle Biodiesel(G3)had less soap and more methanol, indicating a more complete transesterification but more methanol being used by this refinery.The glycerol contents in these crude streams were around55-62%.Glycerol purity in crude glycerol streams has been reported in a wide range from65(7)to85% (26),which probably results from different glycerol purification procedures used by biodiesel plants.The fatty acid compositions of the soap residues were also determined.As shown in Table3.for all three soaps residues, oleic acid(18:1)and linoleic acid(18:2)were the two major fatty acids.G1and G3had very similar fatty acid profiles with high levels of linoleic acid,whereas G2had a relatively higher portion of oleic acid(18:1).These fatty acid profiles were an indication of different feedstocks used in the biodiesel production. Table4shows ICP elemental analysis of the crude glycerol. Potassium was present at high levels in G1and G2,whereas sodium was the major element in G3.This was attributed to the fact that G1and G2came from Virginia Biodiesel using KOH as a catalyst,whereas G3was provided by Seattle Biodiesel utilizing NaOH as a catalyst.Trace amounts of boron, calcium,phosphorus,silicon,and zinc were also found in the crude glycerol samples(Table4).No heavy metals(such as mercury)were found in any of these samples,suggesting crude glycerol can be used as a safe substrate for algal DHA production.Table4also shows that the standard deviations for potassium,silicon,and sodium were relatively high,indicating thefluctuation of these elements in each individual sample. The above results show that the composition of crude glycerol streams varied depending on the feedstocks and biodiesel production processes employed by the refineries.However,all batches of crude glycerol consisted of methanol and soaps as the major impurities.The effects of these two components on DHA production by S.limacinum were further investigated. Effects of Methanol on Algal DHA Production.It has been reported that ethanol is beneficial for the growth of some microalgae producing DHA(27).However,the effect of methanol on algal growth is unknown;this leads us to explore the effects of methanol on DHA production by S.limacinum. The algae were grown in a medium containing pure glycerol (70g/L)with different concentrations of methanol.Considering the fact that methanol evaporates at65°C,the autoclave process (at121°C for15min)resulted in a significant loss of methanol. We did not autoclave the methanol in the medium;instead,theposition of Methanol and Soap Residues in Crude Glycerol Streams acrude glycerol stream composition unit G1G2G3 methanol%,w/w12.79(0.7514.41(2.7028.27(1.99 soap%,w/w25.17(1.7023.24(4.4915.28(3.27 glycerol%,w/w62.04(1.2662.35(3.8956.45(2.98a Data are means of three replicates(standard deviations.Table3.Fatty Acid Composition(Percent of Total Fatty Acid,TFA)of the Soaps asoap sourcefatty acid unit G1G2G316:0%TFA11.61(1.0519.15(2.8211.14(0.00 16:1%TFA ND b 2.70(0.24ND18:0%TFA 4.01(0.89 3.07(2.66 3.61(0.04 18:1%TFA22.97(0.3930.87(3.5923.53(0.08 18:2%TFA54.35(1.4739.58(1.4354.46(0.16 18:3n-3%TFA7.05(0.11 4.63(0.347.25(0.20 TFA mg/g of DW866.46(73.53830.04(59.93895.94(19.49a Data are means of three replicates(standard deviations.b Not detected.Table4.Elemental Composition of Crude Glycerol Streams As Detected by ICP Analysis(Elements Analyzed in Table1But Not Included in This Table Were Not Detected in Any Sample)acrude glycerolelement unit G1G2G3 boron ppm BDL b BDL11.50(0.75 calcium ppm BDL140(36BDL phosphorus ppm BDL480(104BDL potassium ppm31250(21227300(5515BDLsilicon ppm62(2329.80(6.3617.30(2.83 sodium ppm214(45248(4512550(2757 zinc ppm 2.50(0.25 2.30(0.15 2.30(0.15a Data are means of three replicates(standard deviations.b Below detection limit.DHA Production from Biodiesel-Derived Glycerol J.Agric.Food Chem.,Vol.56,No.11,20083935autoclaved medium was spiked with various levels of methanol,sterilized by passage through a 0.2µm filter.As shown in Table 5,the maximum cell dry weight,biomass productivity,and cell yield decreased as methanol concentration increased from 0to 20g/L.In terms of fatty acid composition of the algal biomass,it was found that the proportion of DHA in total fatty acids maintained a relatively constant level;however,the total fatty acid content decreased with methanol concentration (data not shown).This fatty acid profile,together with the cell growth performance,resulted in a decreased DHA production level at higher methanol concentrations.It should be noted that in a previous report (12)in which the methanol effect was studied,methanol was added to the medium and then autoclaved (at 121°C for 15min).We later found that this protocol resulted in a complete loss of methanol within the range of 0–20g/L,as confirmed by HPLC analysis (data not shown).In this study,the sterilization protocols were changed to avoid autoclaving the methanol.Therefore,we believe the results presented in this work reflect the true methanol effects on algal DHA production.The above results clearly indicate the negative effects of methanol on growth and DHA production of S.limacinum .Fortunately,under the typical crude glycerol levels used for algal culture (e.g.,60-90g/L),autoclaving the media can evaporate all the methanol (ca.4–20g/L)contained in the original glycerol stream;this allows the algae to thrive on the methanol-free crude glycerol.Effects of Soap on Algal DHA Production.The effects of soap on algal growth and DHA production were investigated by growing the algal cells in different levels of crude glycerol.The soap residues in the crude glycerol either remained in the medium (soap-containing)or were precipitated into free fattyTable 5.Effects of Methanol Concentration on Growth and DHA Production of S.limacinum (Grown in 70g/L of Pure Glycerol)amethanol concentrationparameter unit 0g/L 10g/L 15g/L 20g/L specific growth rate,µday -10.59(0.010.50(0.030.52(0.030.32(0.01max cell dry wt g/L 11.49(0.4910.31(0.078.36(0.31 5.63(0.47biomass productivity g/L-day 1.92(0.08 1.72(0.01 1.39(0.050.94(0.08growth yield g/g0.23(0.010.23(0.010.18(0.010.13(0.01DHA content mg/g of DW 146.4(6.9158.5(17.7118.7(14.9124.6(2.2DHA yieldg/L 1.68(0.11 1.63(0.180.99(0.130.70(0.06DHA productivityg/L-day0.28(0.020.27(0.030.17(0.020.12(0.01aData are means of three replicates (standarddeviations.Figure 1.Cell growth performance (A -C )and DHA production (D -F )by S.limacinum on soap-free and soap-containing media with different crudeglycerol concentrations.Data are means of three replicates,and error bars show standard deviations.3936J.Agric.Food Chem.,Vol.56,No.11,2008Pyle et al.acids and then removed from the medium(soap-free).The glycerol G1was used as representative of all the crude glycerol samples.The growth kinetics of S.limacinum on soap-containing and soap-free medium were compared.As shown in Figure1A-C in the lower range of crude glycerol concentrations(10and20 g/L),the algal growth rates on soap-free and soap-containing medium were similar.When the crude glycerol concentration increased to40g/L,the specific growth rates of the two cultures were still similar(Figure1A),but the maximum cell dry weight and biomass productivity for soap-containing culture were lower than those for soap-free cultures(Figure1B,C).At60g/Lglycerol concentration,the growth in soap-containing medium was significantly inhibited.It was found that when crude glycerol concentration exceeded60g/L,the algal cells in soap-containing medium were not viable after1day of culture(data not shown).The soap also had significant effects on fatty acid composi-tion.As shown in Table6,in soap-free medium,the proportion of C18fatty acids was negligible(∼1%of TFA),whereas soap-containing medium resulted in significant proportions of C18s fatty acids(25-35%of TFA).Considering the fact that C18s were the major fatty acids contained in soaps(Table3),the difference in fatty acid composition between the soap-free and soap-containing algal biomass indicates that S.limacinum was capable of absorbing those soap C18s into the cells(Table6). However,the proportion of long-chain fatty acids(C22:5and C22:6)in the soap-containing algae was lower than that in the soap-free algae(Table6),suggesting that those absorbed C18s were not further elongated by the algal cells.These fatty acid profiles agree with results reported by Hauvermale et al.(28). These authors used radiolabeled C16:0,C18:1,or C18:3fatty acids to feed Schizochytrium sp.and found that none of the labels appeared in long-chain PUFA(28).Table6also shows that at10g/L of crude glycerol,the algae in soap-containing medium had more total fatty acids(TFAs)than algae in soap-free medium;when crude glycerol concentrations exceeded20 g/L,the TFA content of soap-free algae became more than that of soap-containing algae(Table6).This is probably due to growth inhibition at higher soap levels(Figure1A-C).By combining the soap effects on both cell growth(Figure 1A-C)and fatty acid profile of S.limacinum(Table6),it was found that under different crude glycerol levels,DHA content, yield,and productivity from the soap-containing algae were lower than those obtained from the soap-free algae(Figure 1D-F).The results clearly indicated a negative effect of soap on algal DHA production.This effect is more pronounced at higher levels.The inhibitory effect of soap on S.limacinum agreed with other papers in which the growth of various algal species was inhibited by surfactants including soap(29,30).This inhibition was caused by the complex interaction between the cell wall/ membrane and the surfactants(30).To elucidate the mechanism of soap effects on S.limacinum,further study of the interaction between soaps and cell membrane of this species is needed. Characterization of Algal Biomass Composition.To ensure that crude glycerol-derived algal biomass is suitable for use in omega-3-fortified food or animal feed,a thorough chemical characterization of the algal biomass was performed.Because soap residue had shown negative effects on algal growth and DHA production(Figure1),the algae were grown in soap-free medium.Algae derived from all three crude glycerol samples were evaluated.Algae grown on glucose were also evaluated as a control.Table7shows the proximate analysis of the algal biomass. Overall,the algae grown on the three crude glycerol samples had similar nutritional profiles,except that G2had a relatively high portion of lipid and low portion of protein.The ash content of G1was higher than those of G2and pared with the glucose-derived algae,the glycerol-derived algae had similar lipid and protein contents,but less carbohydrate;the ash content of glycerol algae was higher.Table6.Fatty Acid Composition(Percent of Total Fatty Acid,TFA)of the Algal Biomass Grown in Soap-Free and Soap-Containing Media with Different Crude Glycerol Concentration Loadings aglycerol concentrationfatty acid unit10g/L20g/L40g/L60g/LAlgae on Soap-Free MediumC14:0%TFA 2.06(0.18 3.32(0.24 4.21(0.65 4.77(0.05C16:0%TFA35.50(1.2844.11(0.5349.80(3.7652.64(0.37 C18:0%TFA0.81(0.040.93(0.050.97(0.05 1.04(0.02C22:5%TFA8.58(0.227.35(0.27 6.29(0.77 5.66(0.05C22:6%TFA53.05(1.3044.30(0.5338.73(3.6935.89(0.38 TFA mg/g of DW138.1(2.6292.2(5.9503.9(7.2492.3(12.6Algae on Soap-Containing MediumC14:0%TFA 2.59(0.98 3.14(0.32 2.68(0.25 2.99(0.09C16:0%TFA40.40(12.7342.35(4.2838.35(3.0439.35(0.84 C18:0%TFA 2.33(0.28 1.91(0.50 2.79(1.48 1.10(0.08C18:1%TFA9.17(1.727.43(1.07 6.65(1.00 3.93(0.53C18:2%TFA21.50(6.1117.68(3.2118.77(3.2819.23(1.89 C18:3%TFA 2.81(0.91 2.72(0.61 3.37(0.69 4.85(0.35C22:5%TFA 2.93(0.66 3.55(0.36 3.88(0.49 4.07(0.34C22:6%TFA18.26(4.9421.23(2.0323.50(2.9424.49(2.04 TFA mg/g of DW199.9(62.9282.9(23.1446.2(92.9427.3(71.2a Data are means of three replicates(standard deviations.Table7.Proximate Analysis of Freeze-Dried Algal Biomass Grown onGlucose and Different Crude Glycerol Streams(Soap-free)(75g/L ofCarbon Source Was Used)acarbon sourcecomponent glucose G1G2G3lipid44.61(3.9143.24(1.2850.57(1.3246.71(1.01protein13.05(0.1016.22(0.0013.90(0.0020.78(0.66carbohydrate33.38(3.9125.69(1.3823.93(1.3423.02(1.48ash7.99(0.1413.88(0.5210.62(0.198.51(0.86moisture0.97(0.000.97(0.010.98(0.000.97(0.00a Data(percent)are means of three replicates(standard deviations. DHA Production from Biodiesel-Derived Glycerol J.Agric.Food Chem.,Vol.56,No.11,20083937Table8shows the fatty acid profiles of the algae.All of the carbon sources yield similar fatty acid compositions.C16:0and C22:6(DHA)accounted for about90%of the total fatty acid (TFA).The alga also contained small amounts of C14:0,C18: 0,and C22:5(DPA).The amino acid composition of algal biomass(Table9,left-hand side)is rich in cysteine(4.6-5.2mg/g)and lysine(7.1-8.5 mg/g)relative to many common feedstuffs;lysine and cysteine are frequently thefirst or second limiting amino acid in feedstuffs for a given species(31–33).Although there are significant differences in the concentrations of many amino acids between the algae grown on different carbon sources,this is largely due to differences in overall concentration of protein (Table7).Comparison of the amino acid composition in terms of percent of total protein(Table9,right-hand side)reveals that the compositions of the protein in each alga culture are very similar.Table10shows the results of ICP elemental analysis of algalbiomass.All samples have essentially the same elemental profile. The biomass from G1and G2had higher potassium content than the biomass from G3and glucose,probably due to the high potassium contained in the original G1and G2streams (Table4).Although the stream G3had a higher sodium content than G1and G2(Table4),the algal biomass from these glycerol samples was not significantly different(Table10),due to the fact that the artificial seawater medium contained a high level of sodium.All of the other elements contained in the algal biomass were considered to be resultant from the seawater medium solution,instead of from the crude glycerol.It should be noted that no heavy metals were detected by the ICP analysis.Even specialized testing,using EPA method SW-8467471A with a reporting limit of0.025ppm,did not detect any mercury in the algal biomass.Concerns about heavy metal contamination have limited the usage offish oil products in food or animal feed,but these results alleviate any concerns about heavy metal contamination of algal biomass.In summary,the above compositional analysis indicates that the algal biomass grown in crude glycerol had a similar compositiontothealgaegrowninglucose.Currently,Schizochytri-um algae are available from commercial producers such as Aquafauna Bio-Marine Inc.(Hawthorne,CA;/Profiles-AlgaMac-3000.htm)and AdvancedTable8.Fatty Acid Composition of Algal Biomass Grown on Glucose and Different Crude Glycerol Streams(Soap-free)(75g/L of Carbon Source Was Used)amg/g of dry biomass from carbon source mass%of total fatty acids from carbon sourcefatty acid glucose G1G2G3glucose G1G2G3 C14:033.7(1.028.8(0.629.2(0.331.1(2.387.7(0.2 6.8(0.1 5.9(0.17.0(0.5 C16:0271.2(17.6263.0(1.5287.5(2.2276.1(2.262.2(4.062.2(0.458.4(0.462.1(0.5 C18:0 3.2(0.5 3.3(0.1 4.2(0.1 4.6(1.10.7(0.10.8(0.00.8(0.0 1.0(0.2 C22:58.3(2.79.8(1.214.3(1.211.7(0.8 1.9(0.6 2.3(0.2 2.9(0.2 2.6(0.2 C22:6119.4(13.4117.7(3.1156.9(1.2121.2(2.627.4(3.127.8(0.731.9(0.227.3(0.6a Data are means of three replicates(standard deviations.Table9.Amino Acid Composition of Algal Biomass Grown on Glucose and Different Crude Glycerol Streams(Soap-free)(75g/L of Carbon Source Was Used)amg/g of dry biomass from carbon source mass%of protein from carbon source amino acid glucose G1G2G3glucose G1G2G3 Asx b10.4(0.212.1(1.410.7(0.410.4(0.29.6(0.29.4(1.19.3(0.49.3(0.3 Ser 5.3(0.1 6.2(0.7 5.5(0.3 5.3(0.1 4.9(0.1 4.8(0.5 4.7(0.2 4.7(0.2 Glx b17.2(0.320.5(2.218.8(0.817.2(0.316.0(0.315.9(1.716.2(0.715.3(0.5 Gly 4.4(0.1 5.3(0.7 4.8(0.2 4.4(0.1 4.1(0.1 4.1(0.5 4.1(0.2 4.1(0.1 His 2.4(0.1 2.9(0.4 2.5(0.1 2.4(0.1 2.2(0.1 2.2(0.3 2.2(0.1 2.2(0.1 NH3c 2.2(0.0 2.6(0.1 2.3(0.1 2.2(0.0 2.1(0.0 2.0(0.1 2.0(0.1 1.9(0.2 Arg8.4(0.89.4(0.17.7(0.38.4(0.87.8(0.87.3(0.1 6.7(0.37.5(1.1 Thr 5.4(0.0 6.2(0.7 5.6(0.2 5.4(0.0 5.1(0.0 4.8(0.5 4.8(0.2 4.7(0.2 Ala 6.2(0.17.5(0.9 6.7(0.3 6.2(0.1 5.8(0.1 5.8(0.7 5.8(0.3 5.9(0.2 Pro 4.2(0.0 5.0(0.6 4.4(0.2 4.2(0.0 3.9(0.0 3.9(0.5 3.8(0.2 3.9(0.2 Tyr 4.3(0.3 5.1(0.9 5.4(0.2 4.3(0.3 4.0(0.3 4.0(0.7 4.7(0.2 3.9(0.1 Val 5.8(0.27.0(0.8 6.3(0.3 5.8(0.2 5.4(0.1 5.5(0.6 5.5(0.2 5.5(0.2 Cys 5.2(0.1 4.6(1.6 5.1(0.5 5.2(0.1 4.8(0.1 3.6(1.2 4.4(0.4 4.0(0.1 Met 1.3(1.4 3.9(0.2 3.2(0.3 1.3(1.4 1.2(1.3 3.0(0.1 2.7(0.2 3.4(1.3 Ile 4.6(0.1 5.9(0.7 5.3(0.2 4.6(0.1 4.3(0.1 4.6(0.5 4.6(0.2 4.6(0.2 Leu8.1(0.19.8(1.28.7(0.48.1(0.17.5(0.17.6(0.97.5(0.37.8(0.3 Lys7.1(0.28.5(1.17.5(0.47.1(0.2 6.6(0.1 6.6(0.8 6.5(0.3 6.6(0.2 Phe 5.0(0.1 6.1(0.7 5.4(0.2 5.0(0.1 4.6(0.1 4.7(0.6 4.7(0.2 4.8(0.2a Data are means of three replicates(standard deviations.b Glx)Glu+Gln;Asx)Asp+Asn.c NH3resulted from the deamination of asparagine and glutamine during the analysis process.Table10.ICP Elemental Analysis of Algal Biomass Grown on Glucoseand Different Crude Glycerol Streams(Soap-free)(75g/L of CarbonSource Was Used;Elements Analyzed in Table1But Not Included in ThisTable Were Not Detected in Any Sample)acarbon sourceelement unit glucose G1G2G3boron ppm17.2(5.323.7(4.515.4(1.614.7(0.95calcium ppm506(19603(55647(43592(102copper ppm 6.5(0.9BDL b 4.9(1.8BDLiron ppm69.0(4.2102(4.795.8(8.294.5(5.9magnesium ppm1825(1552050(3351715(2051380(152phosphorus ppm2595(3553740(634290(1143610(124potassium ppm5503(99818600(170012450(11508310(560silicon ppm16.1(3.226.5(3.9519.6(0.410.6(1.74sodium ppm156650(95026400(125014750(175017900(1932sulfur ppm6145(7476530(6057900(6005320(386a Data are means of three replicates(standard deviations.b Below detectionlimit.3938J.Agric.Food Chem.,Vol.56,No.11,2008Pyle et al.。
1GGH安装作业指导书005 (613)

细细胞胞凋亡凋亡和细胞坏死细的胞坏区死
生理性、温和刺激或药物 非同步性、散在、单个细胞
别 病理性、剧烈刺激、损伤
大块组织同时发生
细胞体积缩小
细胞膨胀(内质网、线粒体等)
执行caspase 死亡受体途径的起始caspase 起始caspase
Caspase-12
死亡受体途径的起始caspase
IL-1前体的切割死亡受体途径的起始caspase
Caspase有两类:一类是凋亡起始者,包括 caspase2、caspase8、caspase9;一类是凋亡 执行者,包括caspase3、caspase6.caspase7 起始者负责对执行者的前体进行切割,从而产 生有活性的执行者;执行者负责切割细胞核内 、细胞质中的结构蛋白和调节蛋白。。
Robert Horvitz(罗伯特• 霍维茨)发现了C. elegan中控 制细胞死亡的关键基因(功能性ced-3 和ced-4基因是细 胞死亡执行的一种先决条件, ced-9通过与ced- 3 和ced-4 相互作用阻止细胞死亡), 并描述了其特征他显示了这些 基因在细胞死亡过程中如何互相作用, 并揭示相应的基因 也存在于人体之中。。
Caspase活化
细胞凋亡过程可分为激活期和执行期两个阶段。 前期细胞应答死亡信号, 起始caspase活化;后期 执行caspase活化, 执行细胞死亡程序。 Caspase自身以非活化的Procaspase存在于细胞 质中, 其激活依赖于其他的Caspase在它的天冬氨 酸位点裂解活化或自身活化。 起始Caspase的活化属于同性活化, 执行Caspase 的活化属于异性活化。。
通气培养对小球藻生长及生物组成的影响
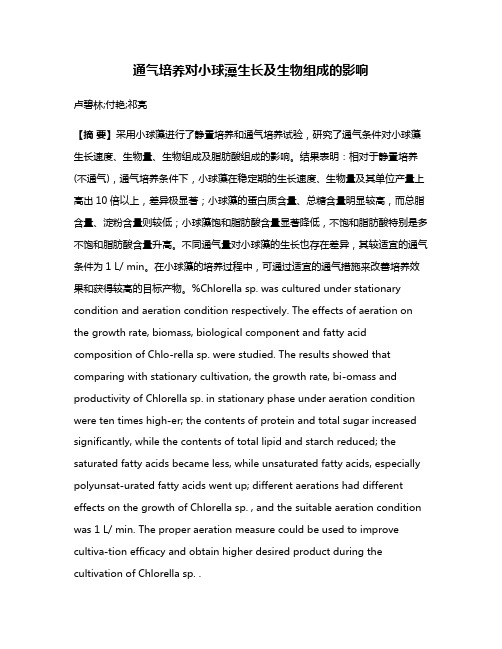
通气培养对小球藻生长及生物组成的影响卢碧林;付艳;祁亮【摘要】采用小球藻进行了静置培养和通气培养试验,研究了通气条件对小球藻生长速度、生物量、生物组成及脂肪酸组成的影响。
结果表明:相对于静置培养(不通气),通气培养条件下,小球藻在稳定期的生长速度、生物量及其单位产量上高出10倍以上,差异极显著;小球藻的蛋白质含量、总糖含量明显较高,而总脂含量、淀粉含量则较低;小球藻饱和脂肪酸含量显著降低,不饱和脂肪酸特别是多不饱和脂肪酸含量升高。
不同通气量对小球藻的生长也存在差异,其较适宜的通气条件为1 L/ min。
在小球藻的培养过程中,可通过适宜的通气措施来改善培养效果和获得较高的目标产物。
%Chlorella sp. was cultured under stationary condition and aeration condition respectively. The effects of aeration on the growth rate, biomass, biological component and fatty acid composition of Chlo-rella sp. were studied. The results showed that comparing with stationary cultivation, the growth rate, bi-omass and productivity of Chlorella sp. in stationary phase under aeration condition were ten times high-er; the contents of protein and total sugar increased significantly, while the contents of total lipid and starch reduced; the saturated fatty acids became less, while unsaturated fatty acids, especially polyunsat-urated fatty acids went up; different aerations had different effects on the growth of Chlorella sp. , and the suitable aeration condition was 1 L/ min. The proper aeration measure could be used to improve cultiva-tion efficacy and obtain higher desired product during the cultivation of Chlorella sp. .【期刊名称】《中国油脂》【年(卷),期】2014(000)008【总页数】3页(P75-77)【关键词】小球藻;通气;生物量;生物组成;脂肪酸组成【作者】卢碧林;付艳;祁亮【作者单位】长江大学农学院,湖北荆州 434025;长江大学地球环境与水资源学院,武汉 430100;长江大学地球环境与水资源学院,武汉 430100【正文语种】中文【中图分类】TS222;Q936藻类具有繁殖快、光合作用效率高、生长周期短、生物质产量高以及不需占用农业用地等优点,同时微藻在生长过程中能吸收利用二氧化碳, 对缓解温室效应具有不可忽视的作用,使得微藻生物质能源开发利用得到了国内外的广泛重视[1-5]。
biodiesel from microagle
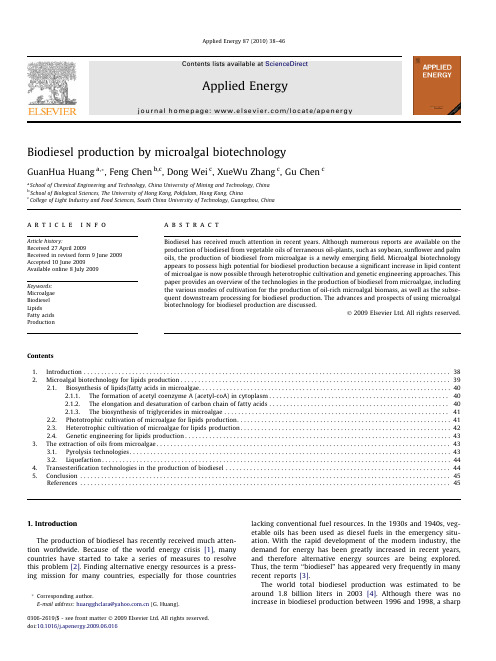
Biodiesel production by microalgal biotechnologyGuanHua Huang a,*,Feng Chen b,c ,Dong Wei c ,XueWu Zhang c ,Gu Chen caSchool of Chemical Engineering and Technology,China University of Mining and Technology,China bSchool of Biological Sciences,The University of Hong Kong,Pokfulam,Hong Kong,China cCollege of Light Industry and Food Sciences,South China University of Technology,Guangzhou,Chinaa r t i c l e i n f o Article history:Received 27April 2009Received in revised form 9June 2009Accepted 10June 2009Available online 8July 2009Keywords:Microalgae Biodiesel LipidsFatty acids Productiona b s t r a c tBiodiesel has received much attention in recent years.Although numerous reports are available on the production of biodiesel from vegetable oils of terraneous oil-plants,such as soybean,sunflower and palm oils,the production of biodiesel from microalgae is a newly emerging field.Microalgal biotechnology appears to possess high potential for biodiesel production because a significant increase in lipid content of microalgae is now possible through heterotrophic cultivation and genetic engineering approaches.This paper provides an overview of the technologies in the production of biodiesel from microalgae,including the various modes of cultivation for the production of oil-rich microalgal biomass,as well as the subse-quent downstream processing for biodiesel production.The advances and prospects of using microalgal biotechnology for biodiesel production are discussed.Ó2009Elsevier Ltd.All rights reserved.Contents 1.Introduction ..........................................................................................................382.Microalgal biotechnology for lipids production ..............................................................................392.1.Biosynthesis of lipids/fatty acids in microalgae.........................................................................402.1.1.The formation of acetyl coenzyme A (acetyl-coA)in cytoplasm ....................................................402.1.2.The elongation and desaturation of carbon chain of fatty acids ....................................................402.1.3.The biosynthesis of triglycerides in microalgae .................................................................412.2.Phototrophic cultivation of microalgae for lipids production..............................................................412.3.Heterotrophic cultivation of microalgae for lipids production.............................................................422.4.Genetic engineering for lipids production.............................................................................433.The extraction of oils from microalgae.....................................................................................433.1.Pyrolysis technologies.............................................................................................433.2.Liquefaction.....................................................................................................444.Transesterification technologies in the production of biodiesel .................................................................445.Conclusion ...........................................................................................................45References ...........................................................................................................451.IntroductionThe production of biodiesel has recently received much atten-tion worldwide.Because of the world energy crisis [1],many countries have started to take a series of measures to resolve this problem [2].Finding alternative energy resources is a press-ing mission for many countries,especially for those countrieslacking conventional fuel resources.In the 1930s and 1940s,veg-etable oils has been used as diesel fuels in the emergency situ-ation.With the rapid development of the modern industry,the demand for energy has been greatly increased in recent years,and therefore alternative energy sources are being explored.Thus,the term ‘‘biodiesel”has appeared very frequently in many recent reports [3].The world total biodiesel production was estimated to be around 1.8billion liters in 2003[4].Although there was no increase in biodiesel production between 1996and 1998,a sharp0306-2619/$-see front matter Ó2009Elsevier Ltd.All rights reserved.doi:10.1016/j.apenergy.2009.06.016*Corresponding author.E-mail address:huangghclara@ (G.Huang).Applied Energy 87(2010)38–46Contents lists available at ScienceDirectApplied Energyj o ur na l h o me pa ge :w w w.e ls e v ie r.c o m/lo c a t e/ap en e rgyincrease in biodiesel production was observed in the past several years.It is speculated that the production of biodiesel will be fur-ther tremendously increased because of increasing demand for fuels and‘‘cleaner”energy globally.Biodiesel is made from biomass oils,mostly from vegetable oils. Biodiesel appears to be an attractive energy resource for several reasons.First,biodiesel is a renewable resource of energy that could be sustainably supplied.It is understood that the petroleum reserves are to be depleted in less than50years at the present rate of consumption[5].Second,biodiesel appears to have several favorable environmental properties resulting in no net increased release of carbon dioxide and very low sulfur content[6,7].The re-lease of sulfur content and carbon monoxide would be cut down by 30%and10%,respectively,by using biodiesel as energy source. Using biodiesel as energy source,the gas generated during com-bustion could be reduced,and the decrease in carbon monoxide is owing to the relatively high oxygen content in biodiesel.More-over,biodiesel contains no aromatic compounds and other chemi-cal substances which are harmful to the environment.Recent investigation has indicated that the use of biodiesel can decrease 90%of air toxicity and95%of cancers compared to common diesel source[64].Third,biodiesel appears to have significant economic potential because as a non-renewable fuel that fossil fuel prices will increase inescapability further in the future[8].Finally,biodie-sel is better than diesel fuel in terms offlash point and biodegrad-ability[9].Conventional biodiesel mainly comes from soybean and vege-table oils[10],palm oil[11],sunflower oil[6],rapeseed oil[12] as well as restaurant waste oil[13].The number of carbon in the carbon chain of the diesel oil molecular is about15,which is similar to that of the plant oil with14–18carbons.The struc-tural characteristic of biodiesel determines that biodiesel is a feasible substitute for conventional energy.Nevertheless,the production cost is generally high for biodiesel.The price of bio-diesel is approximately twofold that of the conventional diesel at present.The production cost of biodiesel consists of two main components,namely,the cost of raw materials(fats and oil) and the cost of processing.The cost of raw materials accounts for60%to75%of the total cost of the biodiesel fuel[14].Though there might be large amounts of low-cost oil and fats available such as restaurant waste and animal fats[15],the major prob-lem of using these low-cost oils and fats is that they often con-tain large amounts of free fatty acids(FFA)which is difficult to convert to biodiesel through transesterification[16].Raw materi-als that contain large proportions of fatty acid triglycerides are preferred.For example,plant oil is found to contain more fatty acid triglycerides and therefore has been used in the production of biodiesel for some years[17].Earlier studies on liquid fuel from microalgae had begun in mid-1980s.During the world war II,although some German scientists attempted to extract lipids from diatom in order to resolve energy crisis[18],and soon later in the USA,research was conducted by a group of scientists at the Carnegie Institution of Washington,and their experiences had been summarized in a book[65]entitled ‘‘Algal Culture from Laboratory to Pilot Plant”,but the technologies of making microalgae as fuels had not been fully exploited.The reasons could be as follows.First,as a source of lipids,microalgae are less known than plants and animals.Second,the prices for most plant oils are relatively low and animal fats are even cheaper; therefore,processes for the microbial oils production have mainly focused on high-valued products that cannot be produced by plants,such as omega-3polyunsaturated fatty acids,especially EPA and DHA[19].In order to resolve the worldwide energy shortage crisis,seek-ing for lipid-rich biological materials to produce biodiesel effec-tively has attracted much renewed interest.Oleaginous microorganisms are favorably considered for their short growth cycles,high lipid contents and ease of being modified by biotech-nological means(see Table1).Some microalgae appear to be suit-able group of oleaginous microorganism for lipids production[20]. Microalgae have been suggested as potential candidates for fuel production because of a number of advantages including higher photosynthetic efficiency,higher biomass production and higher growth rate compared to other energy crops[21–23].Moreover, according to biodiesel standard published by the American Society for Testing Materials(ASTM),biodiesel from microalgal oil is sim-ilar in properties to the standard biodiesel,and is also more stable according to theirflash point values(Table2).2.Microalgal biotechnology for lipids productionMicroalgae have high potentials in biodiesel production com-pared to other oil crops.First,the cultivation of microalgae dose not need much land as compared to that of terraneous plants [20].Biodiesel produced from microalgae will not compromise the production of food and other products derived from crops.Sec-ond,microalgae grow extremely rapidly and many algal species are rich in oils.For instance,heterotrophic growth of Chlorella prototh-ecoides can accumulate lipids as high as55%of the cell dry weight after144h of cultivation with feeding of corn powder hydrolysate in fermenters[24].Oil levels of20–50%are common in microalgae [20].The whole technical process in the production of biodieselTable1Comparison of types of sources for the oils production.Type of organism Advantages DisadvantagesMicroalgal oils(1)Fatty acid constitutions similar to common vegetable oils(1)Most algal lipids have lower fuel value than diesel fuel(2)Under certain condition it may be as high as85%of the dry weight(2)The cost of cultivation is higher compared to common crop oilscurrently(3)Short-time growth cycle(4)Composition is relative single in microalgaeBacteria oils(1)Fast growth rate(1)Most of bacteria can not yield lipids but complicated lipoidOleaginous yeasts and mildews (1)Resources are abundant in the nature(1)Filtration and cultivation of yeasts and mildews with high-content oilsare required(2)High oil content in some species(3)Short-time growth cycle(2)Process of oils extracted from oleaginous yeasts and mildew is complexand new technology should be exploited to resolve it(4)Strong capability of growth in different cultivation on conditions(3)the cost of cultivation is also higher compared to common crop oilscurrently(5)Conversion and utilization of scrapfiber to yield useful oils and theapplication for dealing with waste oils in environmentWaste oils(1)The waste oils is cheap compared to crop oils(1)Containing a lot of saturated fatty acids which is hard to be converted tobiodiesel by catalystG.Huang et al./Applied Energy87(2010)38–4639from microalgae has been well investigated in recent years. Third,the entire production process ranging from the cultivation of high-lipid microalgae to the production of biodiesel from the microalgal oils has also been explored.In the laboratory conditions, the ideal oil content could reach56–60%of total dry biomass by genetic engineering or heterotrophic culture techniques.These technological advances suggest that the industrial production of biodiesel from microalgal oils may be feasible in the near future.2.1.Biosynthesis of lipids/fatty acids in microalgaeIt is known that both inorganic carbon(CO2)and organic carbon sources(glucose,acetate,etc.)can be utilized by microalgae for lip-ids production.The components and contents of lipids in microal-gal cells vary from species to species.The lipid classes basically are divided into neutral lipids(e.g.,triglycerides,cholesterol)and polar lipids(e.g.,phospholipids,galactolipids).Triglycerides as neutral lipids are the main materials in the production of biodiesel.The synthesis routes of triglycerides in microalgae may consist of the following three steps:(a)the formation of acetyl coenzyme A (acetyl-coA)in the cytoplasm;(b)the elongation and desaturation of carbon chain of fatty acids;and(c)the biosynthesis of triglycer-ides in microalgae.2.1.1.The formation of acetyl coenzyme A(acetyl-coA)in cytoplasmThe metabolismflux route on the utilization of carbon dioxide and glucose for the formation of acetyl-coA in microalgae is de-scribed by Yang et al.[25].It is concluded that glyceraldehyde phosphate(GAP)is a key intermediate both for the two metabo-lism systems.The formation of acetyl-coA in photosynthetic reac-tions,including the light reactions,Calvin cycle and synthesis,is located in chloroplast.GAP is withdrawn from Calvin cycle and ex-ported to cytoplasm for consumption.After the export of GAP from chloroplast to cytoplasm,theflow of carbon is directed to the syn-thesis of sugars or oxidation through the glycolytic pathway to pyruvate.Sugars including sucrose are the major storage products in the cytoplasm of plant cells.Akazawa and Okamoto[26]re-ported that glucose was easy to be stored as starch without prior conversion to GAP and then uptake by the chloroplast which sug-gested starch is the main storage formation for carbon source in Chlorella sp.Therefore,one part of the exogenous glucose was di-rectly converted to starch,and the remainder was oxidized through glycolytic pathway.2.1.2.The elongation and desaturation of carbon chain of fatty acidsThe elongation of carbon chain of fatty acids is mainly depen-dent on the reaction of two enzyme systems including acetyl-coA carboxylic enzyme(ACCE)and fatty acid synthase(FAS)in most organisms.In the process of synthesis of fatty acids(Fig.1),acet-yl-coA is the primer.The process of carbon chain elongation needs the cooperation with malonyl-coA,the substrate on which enzyme act are acetyl-ACP and malonyl-ACP.The C16–C18fatty acid thioes-ter can be formed after several reaction steps.The formation of short carbon chain fatty acids is similar in the cells of advanced plants,animals,fungi,bacteria,and algae.For example,in the cell of green algae,the reaction routes of primer such as palmitoleic acid,oleic acid,linoleic acid,linolenic acid in fatty acid synthesis are similar to that in plant cells and yeast cells[27].The desatura-tion of carbon chain of fatty acid occurs from C18and further elon-gation of carbon chain takes place to produce long-chain fatty acids which are unusual in normal plant oils(Fig.2).Long-chain fatty acids(C20–C22)often exist in microalgae and the content varies from species to species[28].Normally,short-chain fatty acids (C14–C18)which are the main components of biodiesel are majority of fatty acids in Chlorella sp.,but high content of long-chain fatty acid and hydrocarbons exist in some specific species of microalgae. So,It is vital to choose proper microalgae species as materials of biodiesel production.Table2Comparison of properties of microalgal oil,conventional diesel fuel,and ASTM biodiesel standard[24].Properties Biodiesel from microalgal oil Diesel fuel ASTM biodiesel standardDensity(kg LÀ1)0.8640.8380.84–0.90Viscosity(mm2sÀ1,cSt at40°C) 5.2 1.9–4.1 3.5–5.0Flash point(°C)11575Min100Solidifying point(°C)À12À50to10–Coldfilter plugging point(°C)À11À3.0(maxÀ6.7)Summer max0Winter max<À15Acid value(mg KOH gÀ1)0.374Max0.5Max0.5Heating value(MJ kgÀ1)4140–45–H/C ratio 1.81 1.81–40G.Huang et al./Applied Energy87(2010)38–462.1.3.The biosynthesis of triglycerides in microalgaeLike other higher plant and animal,microalgae are able to biosynthesize triglycerides to store substance and energy.Gener-ally,L-a-phosphoglycerol and acetyl-coA are two major primers in the biosynthesis of triglycerides.The L-a-phosphoglycerol mainly derives from phosphodihydroxyacetone which is the product of the glycolysis process.The reaction steps are shown in Fig.3.One of the hydroxyl in L-a-phosphoglycerol reacts with acetyl-coA to form Lysophosphatidic acid and later combines with another acetyl-coA to form phosphatidic acid.These two reactions are catalyzed by glycerol phosphate acyl-transferase. In the following steps,lysophosphatidic acid is hydrolyzed by phosphatidate phosphatase to form diglyceride which is then combined with the third acetyl-coA to complete the biosynthesis of triglycerides.The last reaction step is catalyzed by glyceryl diester transacylase.2.2.Phototrophic cultivation of microalgae for lipids productionMicroalgae can transform carbon dioxide from the air and light energy through photosynthesis to various forms of chemical ener-gies such as polysaccharides,proteins,lipids and hydrocarbons. Compared to higher plants,microalgae have a number of advanta-ges including higher photosynthetic efficiency and growth rate [20].In phototrophic culture,usually microalgae can be grown in two systems such as open ponds and enclosed photobioreactors. Enclosed photobioreactor system is more suitable for some micro-algae which are readily contaminated by other microbes,except for some special microalgae which can survive well in extreme envi-ronments such as high pH(e.g.,Spirulina)and high salinity(e.g., Dunaliella)or can grow very rapidly(e.g.,Chlorella)in the open pond.Because of better environmental control,enclosed photobiore-actor system has been suggested for the production of high-value long-chain fatty acids(e.g.,DHA,EPA).Nevertheless,due to the high cost in terms of operation and capital investment and the small scale due to the complexity of bioreactor design compared to open pond system,it might not be economical to produce bio-diesel on a large scale by enclosed photobioreactors.Open pond system is perhaps more suitable for cultivating mic-roalgae for biodiesel because of its relatively cheap operating cost compared to the enclosed photobioreactors.The basic require-ments for microalgal phototrophic growth should include carbon dioxide,other macro-and micro-nutrients,as well as light.Carbon source can be obtained from power plants which release largeG.Huang et al./Applied Energy87(2010)38–4641amounts of waste gases(mainly CO2)daily.Typical coal-fired power plants emit fuel gas from their stacks containing up to 13%CO2.This high concentration of CO2enhances transfer and uptake of CO2in the pond system.The concept of combining a coal-fired power plant with algae cultivation provides a feasible approach to recycle CO2from coal combustion into useable liquid fuel(Fig.4).When grown in large outdoor ponds,microalgae could use CO2from fuel gas directly injected into the culture[29].Be-sides,the wastewater may contain abundant nutrients(e.g.,inor-ganic irons)which are necessary for microalgal growth.Chlorella vulgaris was grown in waste water from a steelmaking plant with the aim of developing an economically feasible system to remove ammonia from wastewater and CO2fromflue gas[30].2.3.Heterotrophic cultivation of microalgae for lipids productionAlthough microalgae can utilize light efficiently,phototrophic growth of microalgae is often slow because of light limitation at high cell densities on a large scale[31]or‘‘photoinhibition”due to excessive light,especially in sunny days[32].In view of these disadvantages associated with photoautotrophic cultivation,het-erotrophic growth of microalgae in conventional fermentors should be favorably considered[33].Heterotrophic cultivation of microalgae offers several advantages over phototrophic cultivation including elimination of light requirement,good control of the cultivation process,and low-cost for harvesting the biomass be-cause of higher cell density obtained in heterotrophic culture of microalgae[34].In heterotrophic culture,both cell growth and biosynthesis of products are significantly influenced by medium nutrients and environmental factors.Carbon sources are the most important element for heterotrophic culture of microalgae in the production of lipids.For example,although the green microalgae C.protothecoides can grow photoautotrophically or heterotrophi-cally.heterotrophic growth of C.protothecoides using acetate, glucose,or other organic compounds as carbon source results in much higher biomass as well as lipid content in cells[35].More-over,heterotrophic microalgae might utilize other carbon sources such as ethanol,glycerol,and fructose depending on the microalgal species used[36].Liu et al.[37]compared several carbon sources and concluded that glucose was preferred.In order to lower the production cost of microalgal oils as biodiesel,cheaper carbon sources should be considered.For instance,corn powder hydroly-sate(CPH)or molasses instead of glucose may be used as organic carbon source in heterotrophic culture.It was reported occasionally CPH was superior to glucose solution,because CPH contained some beneficial components to Chlorella,and as a result,C.protothecoides produced55.2%crude lipids in the cells with a cell dry weight concentration of15.5g LÀ1[24].The utilization of corn powder hydrolysate instead of glucose in heterotrophic culture greatly re-duced the cost of production,which is important for the biodiesel production by microalgae in terms of economical significance.Nitrogen is also an essential macronutrient in lipids plex nitrogen source might be superior to simple nitrogen source in heterotrophic culture of microalgae,because it might provide amino acids,vitamins and growth factors simul-taneously.Industrial wastewater rich in nitrogen also can be con-sidered for the cultivation of microalgae.Monosodium glutamate waste after diluted was well treated as a cheap fermentation medium for Rhodotorula glutinis to biosynthesize lipids as the raw material for the production of biodiesel[38].Many microal-gae growing under nitrogen limitation show enhanced lipid content.In the late1940s,it was noted that nitrogen starvation is most influential on lipid storage and lipid fractions,and as a re-sult of nitrogen starvation,the lipid content as high as70–85%of dry weight was reported[39].It was also reported that Prophyri-dium cruentum might double its total lipid content(mainly neutral lipids)under nitrogen starvation[39].Nevertheless,nitro-gen starvation might not always result in an increase in total lipid content in microalgae but a change in lipid composition.Zhila et al.[40]reported that the alga Botryococcus braunii contained high content(28.4–38.4%)of oleic acid under nitrogen limitation, but the content of total lipids and triacylglycerols did not change.42G.Huang et al./Applied Energy87(2010)38–46It is also noticeable that the overall rates of oil production might be lower in the case of nutrient deficiency due to the overall low-er biomass achieved.Therefore factors other than nitrogen should be considered altogether.Initial carbon to nitrogen(C/N)ratio in the medium has signif-icant impact on the biosynthesis of lipids in microalgae.With the addition of glucose as organic carbon source to the medium and the tremendous decrease of nitrogen source in the medium,a crude lipid content up to55.2%was achieved in heterotrophic C. protothecoides,which was about3.4-fold that in photoautotrophic C.protothecoides[41].Chen and Johns[34]also investigated the ef-fect of C/N ratio and aeration on the fatty acid composition of het-erotrophic Chlorella sorokiniana.When C/N ratio of approximately 20,cell lipid content was at a minimum and increased at both higher and lower C/N values.Environmental factors also cannot be neglected in the growth of microalgae and the formation of fatty acids.The high PUFAs con-tent at low temperature might be explained by the fact that the al-gae need to produce more PUFAs to maintain cell membrane fluidity.Another reason might be that low temperature could lead to high level of intracellular molecular oxygen and hence improves the activities of the desaturase and elongase involved in the bio-synthesis of PUFAs[42].However,the effect of temperature on cell growth and PUFAs production may not be always the same as men-tioned above[31].Therefore,a specific and careful study of the individual microalgae is required.Salinity,pH,and dissolved O2 are also important factors affecting the heterotrophic cultivation of microalgae[43,34].Besides,it has been demonstrated that dif-ferent cultivation modes greatly affect the lipid accumulation in microalgae.Heterotrophically grown microalgae usually accumu-late more lipids than those cultivated photoautotrophically[44].Some phototrophic microorganisms could also be grown on cheap organic substrates heterotrophically[33].Evidences have been shown that the‘‘dark metabolism”of photosynthetic plants and microalgae is similar to that of non-photosynthetic organisms such as yeasts.Recently,an obligate photoautotrophic microalga Phaeodactylum tricornutum was grown heterotrophically when a single gene(Glut1)that encoded the glucose transporter protein (Glut1)was introduced into this alga[45].Such studies on the pro-duction of fatty acids and lipids should be useful for further inves-tigation of microalgae as bio-fuels by heterotrophic cultivation.2.4.Genetic engineering for lipids productionGenetic engineering of microalgae is an upstream technology in microalgal biotechnology.In1960s,cyanobacteria were chosen as ideal material for academic research by scientists.Since the gen-ome of Anabaena PCC7120was successfully cloned,the number of cloned functional genes in cyanobacteria has increased to over 130[46].Acc1is a kind of restriction enzyme which was cloned from oceanic diatom Cylclotella cryptica had been efficiently ex-pressed in C.cryptica for the production of bio-fuel[47].A new method for biodiesel production from microalgal oils has been developed by the application of genetic engineering recently.Na-tional Renewable Energy laboratory in the USA(NREL)has estab-lished engineered microalgae which belong mostly to diatom species.The lipid content of the engineered microalgae increased to above60%in laboratory conditions and above40%in outdoors cultivation,whereas the lipids content in microalgae is5–20%in common natural conditions.The improvement of lipid content in engineered microalgal cells is mainly due to the high expression of acetyl-coA carboxylase gene,which plays an important role in the control of the level of lipid accumulation.At present,the re-search has focused on choosing a proper molecular carrier,making ACC gene full expression in bacteria,yeast,and plant.Furthermore, the modified ACC gene is being introduced into microalgae to ob-tain more efficient expression.The utilization of engineered micro-algae for the production of biodiesel has important economic and environmental benefits.Its superiorities include high yield of mic-roalgae;saving agricultural resources by using seawater as natural medium;the cellular content of lipids in microalgae is several times higher than that in terrestrial plants.3.The extraction of oils from microalgae3.1.Pyrolysis technologiesAlthough oils extracted from microalgal cells have been inves-tigated for fuel production of internal-combustion engines by transesterification of fatty acids[48],industrial biodiesel produc-tion from microalgal oils is still not well developed.At least,high content of microalgal oils is required for this method to realize economic benefits.Since it is difficult to obtain microalgae with high fatty acid content conventionally,it has been considered that only crude fat(lipid)is used for conversion into substitutes for traditional fuels[49].Recent research on the production of energy from renewable sources such as bio-oils production by pyrolysis of biomass has received much interest.Pyrolyzing mic-roalgae to produce liquid fuel wasfirst put forward in Germany in1986.It was reported that the method of catalytic pyrolysis could yield gasoline with high content of aromatic hydrocarbon and octane number[21].Pyrolysis is a phenomenon related to decomposition of biomass under the condition of oxygen defi-ciency and high temperature.Pyrolysis previously wasfirst used for the production of bio-oils or bio-gases from lignocellulose. However,such a technology may be more suitable for microalgae because of the lower temperature required for pyrolysis and the higher-quality oils obtained[50].Moreover,the cost of pyrolysis of lignocellulose is relatively higher than that of microalgae. Compared to lignocellulose,microalgae contain high content of cellular lipids,resolvable polysaccharides and proteins,which are easier to be pyrolyzed to bio-oils and pared to slow pyrolysis[22,23],fast pyrolysis is a new technology, which produces bio-fuel in the absence of air at atmospheric pressure,with a relatively low temperature(450–550°C)and high heating rate(103–104°C sÀ1)as well as short gas residence time to crack into short chain molecules and be cooled to liquid rapidly[50].The main products of slow pyrolysis are char and char-oils with a15–20%yield,whereas,the products of fast pyrolysis are oils and gases with a yield of approximately70% [51].Fast pyrolysis has proved to be a promising way to produce bio-oils compared to slow pyrolysis[41]for the following rea-sons:(1)less bio-oils were produced from slow pyrolysis;(2) the viscous bio-oils from slow pyrolysis is not suitable for liquid fuels;and(3)the fast pyrolysis process is time saving and re-quires less energy compared to the slow prolysis process.Fast pyrolysis tests of microalgae were performed in thefluid bed reactor[52].It was reported that the experiment was completed 500°C with a heating rate of600°C sÀ1,a sweep gas(N2)flow rate of0.4m3hÀ1and a vapor residence time of2–3s.The fast pyrolysis of C.protothecoides and Microcystis aeruginosa yielded 18%and24%of liquid products,pared to the slow pyrolysis from microalgae in autoclave,a great amount of high quality bio-oils can be directly produced from continuously processing microalgae feeds at a rate of4g minÀ1.Many experi-ments have demonstrated that fast pyrolysis(Table3)is an effi-cient method to produce useful fuels and gases from microalgae. As mentioned above,not only the crude fat,but also other chem-ical components such as protein and water-soluble carbohydrate, can be converted easily into fuel oil or gas by thermochemical techniques[53].G.Huang et al./Applied Energy87(2010)38–4643。
拉丁生物制剂命名的基本格式
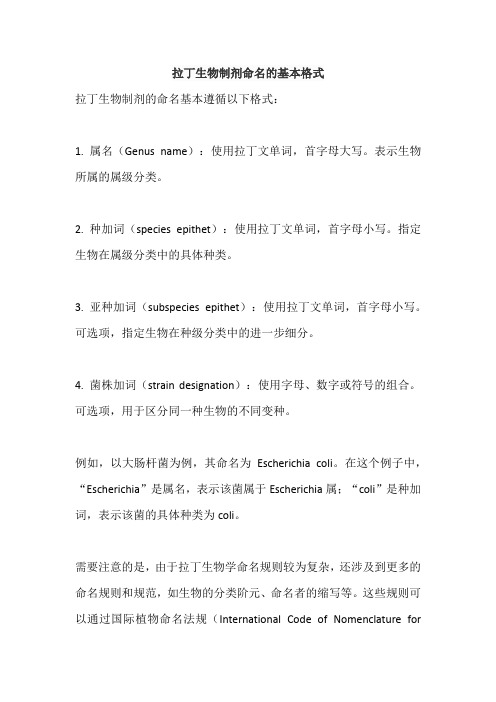
拉丁生物制剂命名的基本格式
拉丁生物制剂的命名基本遵循以下格式:
1. 属名(Genus name):使用拉丁文单词,首字母大写。
表示生物所属的属级分类。
2. 种加词(species epithet):使用拉丁文单词,首字母小写。
指定生物在属级分类中的具体种类。
3. 亚种加词(subspecies epithet):使用拉丁文单词,首字母小写。
可选项,指定生物在种级分类中的进一步细分。
4. 菌株加词(strain designation):使用字母、数字或符号的组合。
可选项,用于区分同一种生物的不同变种。
例如,以大肠杆菌为例,其命名为Escherichia coli。
在这个例子中,“Escherichia”是属名,表示该菌属于Escherichia属;“coli”是种加词,表示该菌的具体种类为coli。
需要注意的是,由于拉丁生物学命名规则较为复杂,还涉及到更多的命名规则和规范,如生物的分类阶元、命名者的缩写等。
这些规则可以通过国际植物命名法规(International Code of Nomenclature for
algae, fungi, and plants)和国际动物命名法规(International Code of Zoological Nomenclature)进行详细了解。
An Overview of Algae Biofuel Production
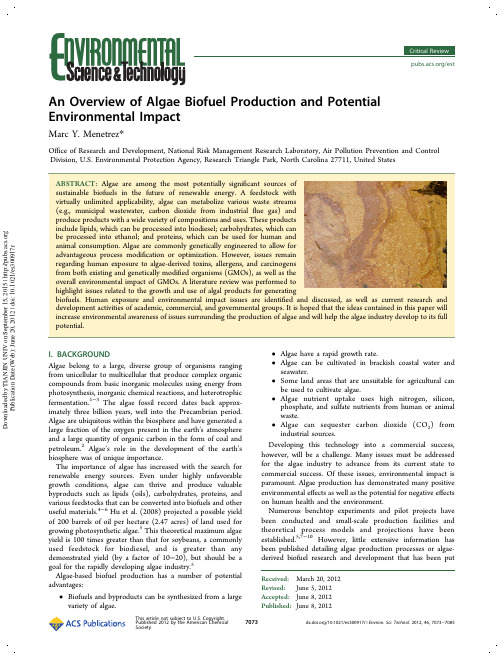
Environmental Science & Technology into practice. Often, the existing literature either is specific to a unique example or is broader in scope. Additionally, what little published information there is often fails to describe the importance of algae production, how that information fits into the present state of knowledge, or what environmental impacts might result from expansion of the algae industry. This paper provides a review of algae generation, the products derived from algae, and the environmental and human health issues surrounding the production process. This review summarizes information related to the status of algae-based biofuel research and development efforts, including the efforts of a number of commercial biofuel companies that are using genetic advancements to move in new directions. These unique genetic advancements are fueling algae biofuel research and making it an ever-changing area of activity with enormous potential environmental consequences. In addition, the risk of exposure to humans and the environment posed by algae biofuel production is summarized and a number of prominent forms of algae production are addressed and compared. 1. Nature of Algae. Algae lack the many distinct organs and structures that characterize land plants, such as leaves, roots, a waxy cuticle, and other organs.1,2 All algae contain green chlorophyll; however, they are masked by photosynthetic pigments that give them a distinguishing color that is used to identify key divisions.1,2 These photosynthetic pigments are made of four different kinds of chlorophyll: blue, red, brown, and gold.1,2 Some algae are microscopic and are able to float in surface waters (phytoplankton) due to their lipid content, while others are macroscopic and attach to rocks or other structures (seaweeds).2 Algae range in size from less than the size of bacteria (0.5 μm) to over 50 m long.2 Algae are cultivated in a variety of aqueous systems, from open air ponds to closed photobioreactors with closely controlled environments.2,11,12 The temperature range needed to support algal growth is specific to the species and strain cultured. The optimal temperature for phytoplankton is within the range of 20−30 °C. Temperatures lower than 16 °C will slow growth, and temperatures higher than 35 °C are normally lethal for a number of species.2,13,14 2. Constituents and Byproducts. The algal growth cycle has demonstrated considerable flexibility in the biochemical ability of algal cells to manufacture various useful compounds. Depending on the species and growing conditions, algae can yield a wide array of byproducts such as lipids, carbohydrates, and proteins.15 Lipids are long carbon chain molecules that serve as a structural component of the algal cell membrane. The increased lipid content decreases the specific gravity, making the algal cell buoyant. The buoyant algal cell then moves up in the water column toward the solar energy source. Algal species vary in lipid production from 20 to 80% (dry weight (DW)). Adjusting the specific species growth requirements can affect the algal biomass lipid, protein, and carbohydrate content.12 These adjustments include environmental culture conditions such as process velocity, length of the growth period, and nutrient supply.15−17 Becker listed the constituents of 17 common algal species.16 The constituents of lipids, starches, and proteins are listed in Table 1. As the table shows, these constituents vary substantially depending on the substrate and environmental conditions of the algal biomass composition.16 Lipids extracted from microalgae can be used to produce biodiesel.12 Once the lipids have been extracted, the leftover solids are composed of mostly carbohydrates and proteins.
生物质转化PPT课件
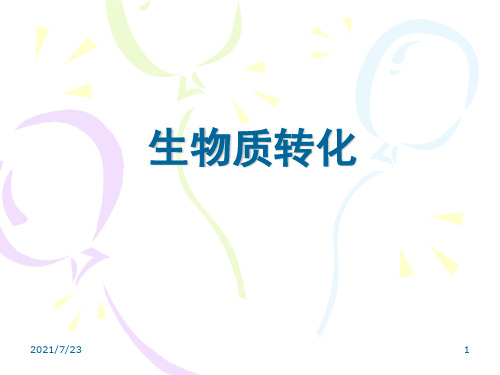
26
2021/7/23
27
生物质化学转化为清洁燃料是生物质能源化利用的重 要技术路线之一
通过生物质直接液化、定向气化、间接液化等生产气 体燃料、液体燃料及含氧燃料(甲醇、二甲醚等)
2021/7/23
化学转化
28
高品位液体燃料
目前石油是我 国高品位液体 燃料主要来源
• 我国人均石油储量不到世界 平均水平的1/10
生物质转化
2021/7/23
1
能源概念和分类
能源是自然界中能为人类提供某种形式能量的物质资 源
按形成条件,能源分为一次能源和二次能源
一次能源指自然界中现成存在,可直接取用的能源
一次能源又可分为可再生能源和非再生能源
二次能源指由一次能源加工转换成的另一种形态的能 能源源按来源分可分为三类
太阳能 地球能 引力能
2.生物质生产的季节性很强。 植物的生长有季节性,难以为化学工业提供稳定的原 料供应,对于现代化学工学的工艺流程提出新的要求
2021/7/23
12
生物质作为化学化工原料的缺点
3.在考虑用作化学化工原料的生物质是传统的粮食, 把粮食原料改作化学化工原料是否合适?
生物质的获取需要土地,大面积的种植对环境有何 影响?
2021/7/23
48
开展微藻生物柴油技术研发的意义
• 开辟一项有长远发展前途的新产业,促进我国相关多学 科的基础研究
• 战略性进入可再生能源领域,从根本上解决“生物原料” 问题
• 减少CO2排放可以进入CDM国际交易,具有商业价值, 这将进一步提高微藻生物柴油技术项目的经济性
• 提前进入温室气体减排运作,2012年中国很可能将正式 承诺减排协议,这意味着项目潜在的经济性巨大
ALGABIO-GB (海藻提取物)

墨角藻
墨角藻是一种褐藻生活在海岸的岩石上。主要生长在布列塔尼海岸,在那里有浓缩海洋活性物。 全年都能收集这种海藻,从它颈部往上20cm用手剪断,以保证可以再次生长。在收割后24小时内进行处理,以保证其新鲜。
润肤 保湿 皮肤保护 再生 补充矿物质 促进内部血液循环 主要活性成分 :
- 多糖: *甘露醇: 是一种有助渗透活性的多羟基化合物 • 海藻酸: 瘦身方面,它是无营养性和卡路里价值的,对皮肤有很好的保湿效果。 *岩藻依聚糖: 有显著的抗凝结特性
岩衣藻
.这种褐藻是在欧洲基石海岸的残骸上发现的。它是一种大型海藻来自Fucale order ,通过它的卵囊来区别的。 整年都能收集到岩衣藻,有1米长,可以通过手来收割。为了能持续收割,只是剪断根部以上20cm的主茎。 只有在收割24小时内转化和加工,才能保证海藻的新鲜。 . 广泛应用于不同领域如海滨疗法,动物饲养和肥料。
各种角叉菜都是天然的美白效果.
润肤剂 保湿 头发护理 收敛 胶凝剂 去角质肥皂剂
主要活性成分: :
- 角叉胶 * 这些分子工业化以后很受欢迎,特别是他们天生能与水形成凝胶或者粘稠状液体
硫酸盐族群的存在赋予了他们抗病毒的特性 * 他们能通过奎宁激活作用阻止血压过低和动脉硬化的发生
* 它们能使蛋白纤维素结合,这种分子结构能提供给表皮良好的保护,对头发或角质细胞有滋润效果
< 100 UFC/g < 10 UFC/g 无 5°C à 25°C 5 kg / 20 kg / 200 kg
水甘油提取物
Nomenclature :
INCI (CTFA)
甘油,水, 墨角藻提取物, 柠檬酸, 苯氧乙醇
* Natural Cosmetics certified by ECOCERT Greenlife according to ECOCERT standard available at :
A_Look_Back_at_the_U.S._Department_of_Energy_s_Aquatic_Species_Program_Biodiesel_from_Algae_July_199

A Look Back at the U.S. Department of Energy’s Aquatic SpeciesProgram—Biodiesel from AlgaeJuly 1998ByJohn SheehanTerri DunahayJohn BenemannPaul RoesslerPrepared for:U.S. Department of Energy’sOffice of Fuels DevelopmentPrepared by: theNational Renewable Energy Laboratory1617 Cole BoulevardGolden, Colorado 80401-3393A national laboratory of the U.S. Department of EnergyOperated by Midwest Research InstituteUnder Contract No. DE-AC36-83CH10093Executive SummaryFrom 1978 to 1996, the U.S. Department of Energy’s Office of Fuels Development funded a program to develop renewable transportation fuels from algae. The main focus of the program, know as the Aquatic Species Program (or ASP) was the production of biodiesel from high lipid-content algae grown in ponds, utilizing waste CO2 from coal fired power plants. Over the almost two decades of this program, tremendous advances were made in the science of manipulating the metabolism of algae and the engineering of microalgae algae production systems. Technical highlights of the program are summarized below:Applied BiologyA unique collection of oil-producing microalgae.either emphasized or characterized algae in terms of these constraints. Early on,researchers set out to build such a collection. Algae were collected from sites in thewest, the northwest and the southeastern regions of the continental U.S., as well aswinnowed down to around 300 species, mostly green algae and diatoms. Thecollection, now housed at the University of Hawaii, is still available to researchers.This collection is an untapped resource, both in terms of the unique organismsavailable and the mostly untapped genetic resource they represent. It is our sincerehope that future researchers will make use of the collection not only as a source ofnew products for energy production, but for many as yet undiscovered new productsand genes for industry and medicine.Shedding light on the physiology and biochemistry of algae.Prior to this program, little work had been done to improve oil production in algalorganisms. Much of the program’s research focused attention on the elusive “lipidand silicon deficiency in diatoms did not turn up any overwhelming evidence inthe cells. The increased oil content of the algae does not to lead to increased overallproductivity of oil. In fact, overall rates of oil production are lower during periods ofnutrient deficiency. Higher levels of oil in the cells are more than offset by lowerrates of cell growth.Breakthroughs in molecular biology and genetic engineering.Plant biotechnology is a field that is only now coming into its own. Within the field of plant biotechnology, algae research is one of the least trodden territories. The slower rate of advance in this field genetics of algae is thus marked with significant scientific discoveries. The program was the first to isolateeventually isolated and cloned. This was the first report of the cloning of the full sequence of the ACCase gene in any photosynthetic organism. With this gene in hand, researchers went on to develop the first successful transformation system for diatoms—the tools and genetic components for expressing a foreign gene. The ACCase gene and the transformation system for diatoms have both been patented. In the gene, a major milestone for the research, with the hope that increasing the level of ACCase activity in the oil production in the cells.Algae Production SystemsDemonstration of Open Pond Systems for Mass Production of Microalgae.Over the course of the program, efforts were made to establish the feasibility of large-scale algae production in open ponds. In studies conducted in California, Hawaii and New Mexico, the ASP proved the concept of long term, reliable production of algae. California and Hawaii served as early test bed sites. Based on results from six years of tests run in parallel in California and Hawaii, 1,000 m2 pond systems were built and tested in Roswell, New Mexico. The Roswell, New Mexico tests proved that outdoor ponds could be run with extremely high efficiency of CO2 utilization. Careful control of pH and other physical conditions for introducing CO2 into the ponds allowed greater than 90% utilization of injected CO2. The Roswell test site successfully completed a full year of operation with reasonable control of the algal species grown. Single day productivities reported over the course of one year were as high as 50 grams of algae per square meter per day, a long-term target for the program. Attempts to achieve consistently high productivities were hampered by low temperature conditions encountered at the site. The desert conditions of New Mexico provided ample sunlight, but temperatures regularly reached low levels (especially at night). If such locations are to be used in the future, some form of temperature control with enclosure of the ponds may well be required.The high cost of algae production remains an obstacle.The cost analyses for large-scale microalgae production evolved from rathersuperficial analyses in the 1970s to the much more detailed and sophisticated studiesconducted during the 1980s. A major conclusion from these analyses is that there islittle prospect for any alternatives to the open pond designs, given the low costrequirements associated with fuel production. The factors that most influence costare biological, and not engineering-related. These analyses point to the need forhighly productive organisms capable of near-theoretical levels of conversion ofsunlight to biomass. Even with aggressive assumptions about biologicalproductivity, we project costs for biodiesel which are two times higher than currentpetroleum diesel fuel costs.Resource AvailabilityLand, water and CO2 resources can support substantial biodiesel production and CO2 savings.The ASP regularly revisited the question of available resources for producing biodiesel from microalgae. This is not a trivial effort. Such resource assessments require a combined evaluation of appropriate climate, land and resource availability. These analyses indicate that significant potential land, water and CO2 resources exist to support this technology. Algal biodiesel could easily supply several “quads” of biodiesel—substantially more than existing oilseed crops could provide. Microalgae systems use far less water than traditional oilseed crops. Land is hardly a limitation. Two hundred thousand hectares (less than 0.1% of climatically suitable land areas in the U.S.) could produce one quad of fuel. Thus, though the technology faces many R&D hurdles before it can be practicable, it is clear that resource limitations are not an argument against the technology.。
杂多酸型离子液体作为温控和环境友好催化剂在微波辅助催化酯化反应

ShortCommunicationA heteropolyacid-based ionic liquid as a thermoregulated and environmentally friendly catalyst in esteri fication reaction under microwave assistanceXixin Duan a ,d ,Guiru Sun a ,Zhong Sun a ,Jianxin Li a ,Shengtian Wang a ,Xiaohong Wang a ,⁎,Shiwu Li b ,Zijiang Jiang caKey Lab of Polyoxometalate Science of Ministry of Education,Northeast Normal University,Changchun 130024,PR China bTraf fic College,Jilin University,Changchun 130025,PR China cChangchun Institute of Applied Chemistry,Chinese Academy of Sciences,Changchun 130022,PR China dFaculty of Chemistry and Biology,Beihua University,Jilin 132013,PR Chinaa b s t r a c ta r t i c l e i n f o Article history:Received 6June 2013Received in revised form 8August 2013Accepted 13August 2013Available online 27August 2013Keywords:Heteropolyacids Ionic liquid Esteri fication Microwave BiodieselA new kind of heteropolyacid (HPA)ionic liquid [(CH 3)3NCH 2CH 2OH]H 2PW 12O 40(ChH 2PW)has been synthe-sized using choline chloride and H 3PW 12O 40(HPW)as precursors.The catalyst exhibited a novel switchable property based on temperature.The separation of the catalyst would be explored by simply decreasing reaction temperature without appreciable loss.Excellent conversions (97%)for esteri fication have been obtained under microwave-accelerated conditions.©2013Elsevier B.V.All rights reserved.1.IntroductionBiodiesel,a mixture of the mono-alkyl esters from esteri fication of fatty acid or transesteri fication of triglyceride molecules,is alternative to conventional diesel fuel [1–3].The routine procedure for biodiesel pro-duction is esteri fication of free fatty acids (FFAs)and transesteri fication of triglycerides [4,5]with short chain alcohols catalyzed by base or acid catalysts [5–7].Heterogeneous acid-catalyzed processes can be applied to produce biodiesel from low-cost raw materials with high amount of FFAs.By now,many approaches involving acid catalysis had been devel-oped [8,9].Compared to homogeneous catalysis,solid acid catalysts have served as important functional materials for green and recyclable catalysts.Heteropolyacids (HPAs)and their salts have been reported as prom-ising heterogeneous catalysts for esteri fication [10–15].Wang Jun's group [16]designed acidic ionic liquid (IL)functional HPAs and acted as a “reaction-induced self-separating catalyst ”for esteri fication.By now,a series of HPA-IL hybrids had been employed as catalysts for es-teri fication and transesteri fication [9,17].Nevertheless,more attentionneeds to be paid for designing new kinds of HPA ILs for esteri fication or transesteri fipared to the most ILs,choline chloride (ChCl)exhibits less expensive,low toxic to humans,environmentally friendly and non-flammable characters [18],which has been used in the conversion of carbohydrates into 5-hydroxymethylfurfural [19,20].The combination of ChCl with Lewis acids had been investigated in o-acetylation of cellulose and monosaccharides [21].As we were preparing our manuscript,Zhang's group [22]reported a series of chorine IL catalysts,which were applied in biodiesel production as ef ficient base catalysts.Therefore,it is bene ficial to replace expen-sive ILs with choline for sustainable synthesis of biodiesel.To our knowledge,there is no report on the combination of ChCl with HPAs on esteri fication.Microwave assisted synthesis of biodiesel showed amazing accelerations,higher yields and high selectivity [23–25].Therefore,we explored an alternative to ful fill esteri fication of FFAs catalyzed by ChCl functionalized HPA catalyst under micro-waves.Its competitive advantages are (1)thermoregulated catalysis.It could be used as a homogeneous catalyst in enhancement of temper-ature.When temperature decreases,it precipitated and could be recycled,just like a heterogeneous system.Thus,this catalyst combines the advantages of homogeneous and heterogeneous catalysis resulting in easy handling of the catalyst;(2)First example of a highly ef ficient use of a ChCl functionalized HPA catalyst;(3)Providing hydrophobic surroundings leading to water-tolerant property availability;(4)VeryCatalysis Communications 42(2013)125–128⁎Corresponding author.Fax:+8643185099759.E-mail address:wangxh665@ (X.Wang).1566-7367/$–see front matter ©2013Elsevier B.V.All rights reserved./10.1016/j.catcom.2013.08.014Contents lists available at ScienceDirectCatalysis Communicationsj o u r n a l h om e p a g e :w w w.e l s e v i e r.c o m /l o c a t e /c a t c o mefficient for the conversion of long chain FFAs;(5)The use of microwave irradiation instead of conventional heating.2.Experimental2.1.Preparation of the catalystsH3PW12O406H2O was synthesized by the reported paper[26]. ChCl(0.467g,3.34mmol)was added to the solution of HPW(10g, 3.34mmol)in20mL of distilled water with stirring at room temperature for8h.A white precipitate was formed and washed with distilled water, recrystallized twice by CH3CN and dried at60°C and then gave a white product[(CH3)3NCH2CH2OH]H2PW12O40(ChH2PW)with the yield of 71.2%.[(CH3)3NCH2CH2OH]2HPW12O40abbreviated as(Ch)2HPW was prepared by the similar procedure with the molar ratio of ChCl to H3PW12O40as2:1.[C16H33N(CH3)3]H2PW12O40(CTAH2PW)was pre-pared by the previous report[27].2.2.Esterification reactionEsterification reactions were carried out in the presence of ChH2PW at different reaction conditions.This reaction mixture was then irradiat-ed by microwave(A2450MHz microwave generator)under reflux (65°C)for the specified reaction time.After the esterification reaction completed,the upper layer were esters and the excess alcohol and the lower layer was the catalyst,which was recovered by decantation and washing with methanol and removing water under a vacuum at60°C for further use.The mixture was concentrated using a rotary evaporator to remove the excess alcohol.The conversion of free fatty acid into ester was calculated by measuring the acid value of the product and the yield of ester was detected by gas chromatography(GC).3.Results and discussion3.1.Catalyst characterizationThe FT-IR spectrum of ChH2PW was given in Fig.S1a.The four bands at1080,978,893and808cm−1are due to v as(P–O a),v as(W–O d), v as(W–O b)and v as(W–O c),respectively,corresponding to the charac-teristic bands of Keggin structure[18].It indicated that the catalyst well retained the Keggin structure after the organic cation replaced the proton in HPW.The peaks at3519,3037and1471cm−1were at-tributed to C\H,and C\N,respectively,showing the existence of the organic group.The X-ray diffraction pattern was used to confirm the structure of pared with pure HPW,ChH2PW shows the similar diffrac-tion peaks(Fig.S2)at10°,15–18°,21–27°and30–40°(JCPDS no.76-1815).This result indicates the original structure of HPW being attained after forming HPAs.The31P MAS NMR spectrum of ChH2PW(Fig.S3)shows one peak at δ=−16.2ppm,whereas H3PW12O40·6H2O gives peak at−15.6ppm. The shift of31P MAS NMR is attributed to the introduction of organic cations into HPW,which could confirm the formation of ChH2PW and no physical mixture of ChCl and PW12O403−.The transmission electron microscopy(TEM)image(Fig.1)shows that the catalyst consists of irregular particles with sizes from10to 20nm.The result of energy-dispersive X-ray(EDX)measurement suggested the molar ratio of C:P:W=5:1:12,giving the formula as [(CH3)3NCH2CH2OH]H2PW12O40.These results demonstrate the forma-tion of ChH2PW(Scheme S1).Also,the elemental analyses of Ch2HPW are:W,72.8;P,1.11;C,2.10;H,0.65;and N,0.55%,respectively.The formation of micellar assembly of ChH2PW had been deter-mined using specific conductivity to define the critical micelle concen-tration(CMC)of ChH2PW(Fig.S4).It gave nearly a portion of two straight lines with break points of specific conductivity versus concen-tration plot[28].Therefore,ChH2PW is a kind of amphiphilic molecules, which could form micellar assembly in water or polar-solvent.The Brønsted acidity of ChH2PW was determined by titration [29].The acidity capacity of ChH2PW is about0.62mmol/g,which is lower than that of HPW(1.79mmol/g),but higher than that of ChCl (0.02mmol/g).This could be attributed to the organic fragment instead of the proton of H3PW12O40.3.2.The catalytic activity of ChH2PWIn esterification of palmitic acid and methanol by microwave assis-tance,different catalysts gave various performances,while the catalytic activity(Fig.2)was in the range of HPW~ChH2PW N ChCl.HPW as a homogeneous catalyst,presented the conversion of98.5%and TOF of 47.2h−1.ChH2PW exhibited the similar performance as homogeneous HPW.This result is very important that there are only a few successful examples at present,in which the catalytic activity of heterogeneous HPAs is comparable to those of the homogeneous analogs[30].Com-pared to other HPAs supported on silica,our results showed the higher activity with lower usage of methanol and short reaction time[31]due to the unique properties of ChH2PW.The higher activity of ChH2PW comes from the acid center of H2PW12O40−and thermoregulated property of cholinefunctionalizedFig.1.The TEM image(left)and EDX pattern(right)of ChH2PW.126X.Duan et al./Catalysis Communications42(2013)125–128HPA (Fig.3).It can be seen that before the reaction,ChH 2PW decanted to the bottom of the reactor to form heterogeneous one with methanol (Fig.3a).With an increase in temperature to 65°C,ChH 2PW dispersed around methanol phase (Fig.3b).The morphology of this form was test-ed by TEM (Fig.1),showing that ChH 2PW assembles as micellar spheres with size around nanometers.In addition,the formation of micellar assembly in methanol at 65°C had been con firmed by the CMC test.Therefore,choline functionalized HPAs could act as a temperature-responsive catalyst in this reaction.The catalytic activity of ChH 2PW could be enhanced and exhibited a high conversion of 97%,which is close to that of HPW.After the reaction,with a decrease in temperature to the room temperature,the catalyst precipitated from the mixture to be separated for reuse.In addition,microwave assistance (97%for 50min)is more available for esteri fication and can reduce reaction time compared to the conventional heating (96.5%for 5.5h and 52.5%for 50min).Therefore,microwave assistance could promote the reac-tion and shorten the reaction time.Another purpose of this work is to seek a heterogeneous acid catalyst to ful fill esteri fication of FFAs in crude feedstocks containing water.The presence of water could promote the reverse reaction,therefore the effect of water on catalytic activity had been done by adding someexcess water to the mixture.It can be seen that the conversion was related to water content (Fig.4).When water content was below 0.2%,the conversion was reduced to 72.8%,but the conversion was signi fi-cantly reduced to 1.46%for 1%of water.This result is comparable to the other solid HPA catalyst Cs 2.5H 0.5PW 12O 40[32]and higher thanH 2SO 4[33]and SO 42−/ZrO 2[34].The reason is that ChH 2PW is composed of choline hydrophobic tail and a HPA hydrophilic head group,whose hydrophobic tail would help water molecules be eliminated from the catalytic pared to the other amphiphilic HPA molecules such as CTAH 2PW (CTA represents cetyltrimethyl ammonium),the longer organic groups led to higher water-tolerance.The in fluence of chain length of acid and alcohol on esteri fication with ChH 2PW had been checked.The conversions for different alcohols (methanol,ethanol,and butanol)are shown in Fig.S5.The type of alcohols affects the reaction very obviously,which is perhaps because of the different dissociation degree of three alcohols.It is known that for alcohols,the acidity decreased as the alkyl group substitution increased [35]resulting in weak dissociation degree.Therefore,metha-nol can be easier to form alkoxide ions compared with other alcohols,which is helpful for esteri fication.Therefore,the different acids reacting with methanol give the highest conversion compared to other alcohols and the different lengths of acid did not show signi ficant in fluence on esteri fication.As a heterogeneous acid catalyst,ChH 2PW could form micellar assembly in methanol during the reaction.The acid sites from HPAs are fixed on the surface of the assembly,and the palmitic acid molecules are easy to access to the catalytic sites.Therefore,no steric effects were observed in esteri fication of different carboxylic acids.Main parameters affecting esteri fication were investigated in Fig.S6.The optimism reaction conditions are the molar ratio of methanol/acid/catalyst 520:40:1,reaction time 50min and temperature 65°C.As for heterogeneous catalysts,their regeneration is the most impor-tant parameter in application.This thermoregulated ChH 2PW can be easily achieved by decreasing the temperature (Fig.3c).The catalytic activity remains ef ficient after six runs,showing only a slight decrease in activity (Fig.5).The leaching of ChH 2PW was about 1.1wt.%for each cycle.And the total amount of ChH 2PW leaching through six runs is 6.5%of the starting amount.In order to determine the leaching of ChH 2PW,the UV –vis spectroscopy of the sample in methanol was done.The two characteristic bands at 221nm and 260nm could be seen corresponding to the charge transfer of oxygen to tungsten,indi-cating that ChH 2PW dissolved in methanol with Keggin structure.It can be concluded that the leaching of ChH 2PW was attributed to the dis-solution in methanol during the reaction.The IR spectrum of ChH 2PW20406080100120C h H 2P W (b )C h H 2P W (c )(C h )2H P W (a )C h H 2P W (a )C h C l (a )H P W (a )T O F (h -1) a n d C o n v e r s i o n (%)Fig.2.Esteri fication of palmitic acid with different catalysts.Reaction conditions:palmiticacid0.02mol,the molar ratio of methanol/acid/catalyst 520:40:1,50min,65°C,(a)micro-wave irradiation,50min;(b)conventional heating,50min;(c)conventional heating,5.5h.Fig.3.Photographs of the esteri fication of palmitic acid and methanol over ChH 2PW.(a)Before the reaction;(b)homogeneous mixture during the reaction;(c)after the reaction.020406080100C o n v e r s i o n (%)content of water (%)Fig.4.Effect of water on the esteri fication.Reaction conditions:palmitic acid 0.02mol,the molar ratio of methanol/acid/catalyst 520:40:1,50min,65°C.127X.Duan et al./Catalysis Communications 42(2013)125–128after esteri fication (Fig.S1b)con firmed that the catalyst maintained its Keggin structure.In addition,the nature of ChH 2PW had been tested.ChH 2PW was in contact with palmitic acid at 65°C during 50min and afterwards the solid was separated from the liquid phase by decanta-tion;then,methanol was added to the liquid phase and the reaction was monitored during 50min.The conversion was only 20.3%.The compared experiment had been done,while the catalyst was in contact with methanol in similar condition then reacted with palmitic acid.The conversion was about 21.1%.From the above result,ChH 2PW was con firmed as a heterogeneous one with a little leaching amount.4.ConclusionsChH 2PW had been fabricated by ChCl and HPW which results in nonconventional IL HPAs.It was used as a “temperature controlled self-separation catalyst ”for esteri fication and made the recovery and reuse very convenient.Microwave assistance greatly shortened the reaction time and was successfully contributed to esteri fication with high ef ficiency.It is an important result switching from homogeneous to heterogeneous catalysis system.AcknowledgmentsThe work was supported by the National Natural Science Foundation of China (51078066and 20871026)and the project of Jilin ProvincialScience and Technology Department (20086035,20100416,and 201105001).Appendix A.Supplementary dataSupplementary data to this article can be found online at /10.1016/j.catcom.2013.08.014.References[1]M.E.Borges,L.Díaz,Renew.Sustain.Energy Rev.16(2012)2839–2849.[2]T.M.Mata,I.R.B.G.Sousa,S.S.Vieira,N.S.Caetano,Energy Fuel 26(2012)3034–3041.[3] A.Talebian-Kiakalaieh,N.A.S.Amin,H.Mazaheri,Appl.Energy 104(2013)683–710.[4]W.Chen,P.Yin,H.Chen,Z.Wang,Ind.Eng.Chem.Res.51(2012)5402–5407.[5] B.Zhen,H.Li,Q.Jiao,Y.Li,Q.Wu,Y.Zhang,Ind.Eng.Chem.Res.51(2012)10374–10380.[6] A.Baig,F.T.T.Ng,Energy Fuel 24(2010)4712–4720.[7]G.W.Tan Zillillah,Z.Li,Green Chem.14(2012)3077–3086.[8] A.Patel,N.Narkhede,Energy Fuel 26(2012)6025–6032.[9]H.Li,Y.Qiao,L.Hua,Z.Hou,B.Feng,Z.Pan,Y.Hu,X.Wang,X.Zhao,Y.Yu,ChemCatChem 2(2010)1165–1170.[10]W.H.Zhang,Y.Leng,D.R.Zhu,Y.J.Wu,J.Wang,mun.11(2009)151–154.[11] C.F.Oliveira,L.M.Dezaneti,F.A.C.Garcia,J.L.de Macedo,J.A.Dias,S.C.L.Dias,K.S.P.Alvim,Appl.Catal.A Gen.372(2010)153–161.[12]Y.Leng,J.Wang,D.R.Zhu,Y.J.Wu,P.P.Zhao,J.Mol.Catal.A Chem.313(2009)1–6.[13]L.H.Wee,S.R.Bajpe,N.Janssens,I.Hermans,K.Houthoofd,C.E.A.Kirschhock,J.A.Martens,mun.46(2010)8186–8188.[14]L.L.Xu,Y.H.Wang,X.Yang,X.D.Yu,Y.H.Guo,Green Chem.11(2009)314–317.[15]V.Brahmkhatri,A.Patel,Appl.Catal.A Gen.403(2011)161–172.[16]Y.Leng,J.Wang,D.R.Zhu,X.Q.Ren,H.Q.Ge,L.Shen,Angew.Chem.Int.Edit.48(2009)168–171.[17]Y.Qiao,Z.Hou,.Chem.13(2009)1347–1365.[18] F.Ilgen,D.Ott,D.Kralisch,C.Reil,A.Palmbergera,B.König,Green Chem.11(2009)1948–1954.[19]S.Q.Hu,Z.F.Zhang,Y.X.Zhou,J.L.Song,H.L.Fan,B.X.Han,Green Chem.11(2009)873–877.[20] F.Liu,J.Barrault,K.D.Oliveira Vigier,F.Jérôme,ChemSusChem 5(2012)1223–1240.[21] A.P.Abbott,T.J.Bell,S.Handa,B.Stoddart,Green Chem.7(2005)705–707.[22]M.M.Fan,J.L.Huang,J.Yang,P.B.Zhang,Appl.Energy 108(2013)333–339.[23] D.Kim,J.Choi,G.J.Kim,S.K.Seol,S.Jung,Bioresour.Technol.102(2011)7229–7231.[24] D.Kim,J.Choi,G.J.Kim,S.K.Seol,Y.C.Ha,M.Vijayan,S.Jung,B.H.Kim,G.D.Lee,S.S.Park,Bioresour.Technol.102(2011)3639–3641.[25]H.Zhang,J.Ding,Z.Zhao,Bioresour.Technol.123(2012)72–77.[26]R.C.Deltcheff,M.Fournier,R.Franck,R.Thouvenot,Inorg.Chem.22(1983)207–216.[27]M.X.Cheng,T.Shi,H.Y.Guan,S.T.Wang,X.H.Wang,Z.J.Jiang,Appl.Catal.B Environ.107(2011)104–109.[28]N.Nazir,M.S.Ahanger,A.Akbar,J.Dispers.Sci.Technol.1(2009)51–55.[29]L.N.Zhou,K.Liu,W.M.Hua,Y.H.Ying,Z.Gao,Chin.J.Catal.30(2009)196–200.[30]N.Mizuno,K.Kamata,Coord.Chem.Rev.255(2011)2358–2370.[31] B.Zhen,H.S.Li,Q.Z.Jiao,Y.Li,Q.Wu,Y.P.Zhang,Ind.Eng.Chem.Res.51(2012)10374–10380.[32]F.Chai,F.H.Cao,F.Y.Zhai,Y.Chen,X.H.Wang,Z.M.Su,Adv.Synth.Catal.349(2007)1057–1065.[33] D.Kusdiana,S.Saka,Bioresour.Technol.91(2004)289–295.[34]J.Jitputti,B.Kitiyanan,P.Rangsunvigit,K.Bunyakiat,L.Attanatho,P.Jenvanitpanjakul,Chem.Eng.J.116(2006)61–66.[35]J.A.Colucci,E.E.Borrero,F.Alape,J.Am.Oil Chem.Soc.82(2005)525–530.102030405060708090100012345678910L e a c h i n g o f c a t a l y s t (w t %)C o n v e r s i o n (%)RunsFig.5.The reuse of the catalyst (palmitic acid 0.02mol,the molar ratio of methanol/acid/catalyst 520:40:1,50min,65°C).128X.Duan et al./Catalysis Communications 42(2013)125–128。
对生物药的热爱作文

对生物药的热爱作文英文回答:I have always had a passion for biologics. The way these drugs are developed using living organisms like bacteria or yeast fascinates me. The complexity and precision involved in creating these medications to treat various diseases is truly remarkable.One of the reasons I love biologics is because of their specificity. Unlike traditional small molecule drugs, biologics target specific proteins or cells in the body, which can lead to more targeted and effective treatments with fewer side effects. For example, monoclonal antibodies are a type of biologic drug that can specifically bind to certain proteins on cancer cells, helping to stop their growth and spread.Another reason I am drawn to biologics is their potential for personalized medicine. With advancements inbiotechnology, it is now possible to tailor biologic treatments to individual patients based on their genetic makeup or the specific characteristics of their disease. This personalized approach can lead to better outcomes for patients and a more efficient use of healthcare resources.In addition, I am inspired by the innovation and creativity that goes into developing biologics. Scientists and researchers are constantly pushing the boundaries of what is possible in medicine, finding new ways to harnessthe power of living organisms to improve human health. The process of discovering, developing, and bringing a biologic drug to market is a long and challenging journey, but the potential impact on patients' lives makes it all worthwhile.Overall, my love for biologics stems from theincredible science behind these drugs, their potential for personalized medicine, and the innovation that drives the field forward. I am excited to see what the future holdsfor biologics and how they will continue to revolutionizethe way we treat diseases.中文回答:我一直对生物药充满热爱。
基于尼罗红荧光染色的小球藻脂质快速检测方法研究_王海英
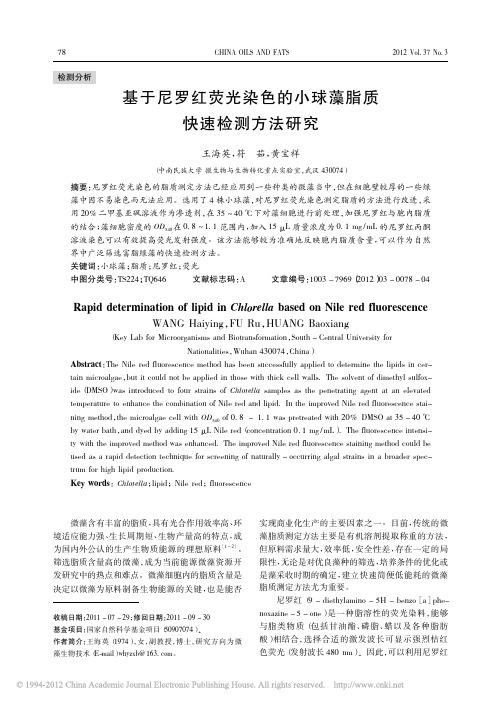
除了特殊说明,以下所有实验均做 3源自个平行。 1. 4 称重法[6]提取溶剂为氯仿 - 甲醇混合溶液 ( 体积比为 2 ∶ 1) ,室温下在 0. 3 g 藻粉中加入 3 mL 提取溶剂, 超声提取约 10 min,6 000 r / min 离心 5 min,取固体 层以下脂质层,重复此步骤 3 ~ 5 次。合并所有脂质 层溶液于已恒重好的离心管中,于 80 ℃ 水浴蒸干溶 剂,放入 40 ℃ 烘 箱 中 过 夜 干 燥 至 恒 重,计 算 脂 质 含量。 1. 5 数据处理
藻中因不易染色而无法应用。选用了 4 株小球藻,对尼罗红荧光染色测定脂质的方法进行改进,采
用 20% 二甲基亚砜溶液作为渗透剂,在 35 ~ 40 ℃ 下对藻细胞进行前处理,加强尼罗红与胞内脂质
的结合; 藻细胞密度的 OD540 在 0. 8 ~ 1. 1 范围内,加入 15 μL 质量浓度为 0. 1 mg / mL 的尼罗红丙酮 溶液染色可以有效提高荧光发射强度。该方法能够较为准确地反映胞内脂质含量,可以作为自然
78 检测分析
CHINA OILS AND FATS
2012 Vol. 37 No. 3
基于尼罗红荧光染色的小球藻脂质 快速检测方法研究
王海英,符 茹,黄宝祥
( 中南民族大学 微生物与生物转化重点实验室,武汉 430074)
摘要: 尼罗红荧光染色的脂质测定方法已经应用到一些种类的微藻当中,但在细胞壁较厚的一些绿
界中广泛筛选富脂绿藻的快速检测方法。
关键词: 小球藻; 脂质; 尼罗红; 荧光
中图分类号: TS224; TQ646
文献标志码: A
文章编号: 1003 - 7969( 2012) 03 - 0078 - 04
Rapid determination of lipid in Chlorella based on Nile red fluorescence WANG Haiying,FU Ru,HUANG Baoxiang
生物柴油讲义

Chemical Reaction
催化剂
biodiesel By trans-esterification, fatty acid methyl esters known as biodiesel originating from algal oils were produced.
异养藻粉
异养藻油脂
生物柴油
生物柴油成分GC-MS分析
GC
N o. Molecula r formula Relative molecul ar mass 242 270 284 294 296 298 310 324 326
MS
Fatty acid methyl ester Relative content (%)
Strain (%)
Protein (%)
Lipid (%)
Carbohydrate (%)
Ash (%)
Moisture (%)
Others (%)
AC
52.64
14.57
10.62
7.38
8.74
6.05
HC
10.28
54.70
15.19
7.24Βιβλιοθήκη 5.407.19Cell acquisition and lipid extraction
研究方案框图
诱变筛选 异养转化 C. protothecoides C. protothecoides C. protothecoides 自养细胞 异养细胞 “三高藻种” 比 关键 酶 代谢 通量 较 蛋白 组学 分 油脂 特征 析 脂肪酸 组成
目标:
油脂富集机理
油脂积累调控规律
油脂含量 占用土地 发展空间
很少 潜力大 (研究工作刚开始)
小头端菱形藻生长的最适光照强度及氮磷比
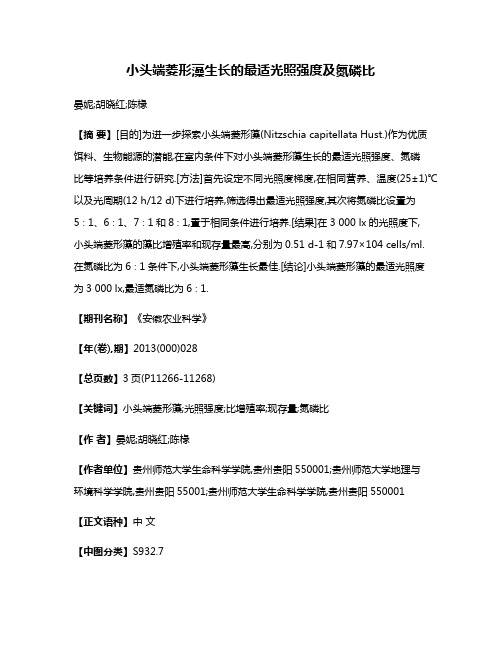
小头端菱形藻生长的最适光照强度及氮磷比晏妮;胡晓红;陈椽【摘要】[目的]为进一步探索小头端菱形藻(Nitzschia capitellata Hust.)作为优质饵料、生物能源的潜能,在室内条件下对小头端菱形藻生长的最适光照强度、氮磷比等培养条件进行研究.[方法]首先设定不同光照度梯度,在相同营养、温度(25±1)℃以及光周期(12 h/12 d)下进行培养,筛选得出最适光照强度,其次将氮磷比设置为5∶1、6∶1、7∶1和8∶1,置于相同条件进行培养.[结果]在3 000 lx的光照度下,小头端菱形藻的藻比增殖率和现存量最高,分别为0.51 d-1和7.97×104 cells/ml.在氮磷比为6∶1条件下,小头端菱形藻生长最佳.[结论]小头端菱形藻的最适光照度为3 000 lx,最适氮磷比为6∶1.【期刊名称】《安徽农业科学》【年(卷),期】2013(000)028【总页数】3页(P11266-11268)【关键词】小头端菱形藻;光照强度;比增殖率;现存量;氮磷比【作者】晏妮;胡晓红;陈椽【作者单位】贵州师范大学生命科学学院,贵州贵阳550001;贵州师范大学地理与环境科学学院,贵州贵阳55001;贵州师范大学生命科学学院,贵州贵阳550001【正文语种】中文【中图分类】S932.7光对植物生长和发育的重要性不言而喻。
除了作为光合作用能源外,光控制植物(包括藻类)的一系列发育过程即光形态建成[1]。
藻类的许多发育过程如营养、生殖结构的形成等受光的影响。
除光照外,在众多环境因素中,氮、磷2种元素被认为是影响藻类生长的重要限制因子。
氮和磷的作用是相互影响的。
不同的藻类对氮磷的需求量不同。
目前,已有很多单细胞绿藻(如小球藻属)和甲藻的研究资料,但对淡水和海洋中的重要浮游植物——硅藻仍缺少研究[2]。
浮游硅藻作为一类重要的微藻,其营养丰富,易被消化,是滤食性动物的重要天然饵料[3],尤其菱形藻属(Nitzschia)中许多种类是水产养殖上具有较大经济价值的优良藻种[4-5]。
三角褐指藻(Phaeodactylum tricornutum)通用转化载体的构建

三角褐指藻(Phaeodactylum tricornutum)通用转化载体的构建郑国庭;姜鹏;秦松;严小军【摘要】Phaeodactylum tricornutum is an ideal material for biodiesel research. In this paper, several endogenous elements, i.e. promoters and terminators, fromfcp (fucoxanthin, chlorophyll a/c-binding protein) gene clusters were cloned, and a transformation vector pfcpA-MCS/fcpB-bar including two expression cassettes which were fcpB promoter-bar gene-fcpA terminatiom and fcpA promoterm-MCS (multiple cloning site)-fcpA terminatiom was constructed and characterized by using bar gene as selection marker. It carried a large MCS easy for insertion of one or more function genes. This vector will be used for lipid metabolic engineering for Phaeodactylum tricorn-utum.%三角褐指藻(Phaeodactylum tricornutum)是开展微藻生物柴油研究的理想材料.克隆了内源fcp基因簇的多个调控序列(启动子、终止子),构建了包括fcpB启动子-bar基因-fcpA终止子、以及fcpA启动子-多克隆位点(MCS)-fcpA终止子两个表达盒的通用转化载体pfcpA-MCS/fcpB-Bar,其特征是以bar基因作为选择标记,MCS区方便插入一至多个目的基因.新载体可用于三角褐指藻的重组蛋白表达、或油脂代谢相关基因的功能验证和代谢调控研究.【期刊名称】《生物学杂志》【年(卷),期】2012(029)004【总页数】4页(P8-11)【关键词】bar基因;三角褐指藻;通用转化载体【作者】郑国庭;姜鹏;秦松;严小军【作者单位】宁波大学,浙江省海洋生物工程重点实验室,宁波,315211;中国科学院海洋研究所,青岛,266071;中国科学院海洋研究所,青岛,266071;宁波大学,浙江省海洋生物工程重点实验室,宁波,315211【正文语种】中文【中图分类】Q943.2三角褐指藻(Phaeodactylum tricornutum Bohlin)属羽纹纲海洋硅藻,富含蛋白、多糖、多不饱和脂肪酸等多种高值活性物质,是人工育苗的常规优良饵料[1]。