Carbon 03-S urface modification of a granular activated carbon by citric
石墨相氮化碳光催化还原CO2研究进展
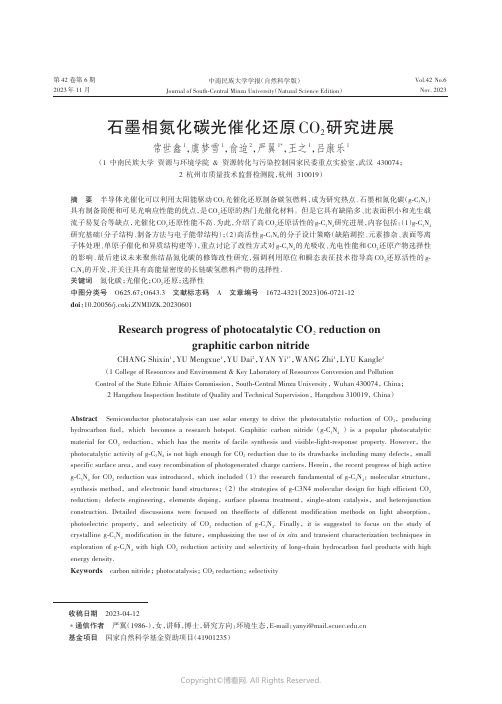
第 42 卷第 6 期2023年 11 月Vol.42 No.6Nov. 2023中南民族大学学报(自然科学版)Journal of South-Central Minzu University(Natural Science Edition)石墨相氮化碳光催化还原CO2研究进展常世鑫1,虞梦雪1,俞迨2,严翼1*,王之1,吕康乐1(1 中南民族大学资源与环境学院& 资源转化与污染控制国家民委重点实验室,武汉430074;2 杭州市质量技术监督检测院,杭州310019)摘要半导体光催化可以利用太阳能驱动CO2光催化还原制备碳氢燃料,成为研究热点.石墨相氮化碳(g-C3N4)具有制备简便和可见光响应性能的优点,是CO2还原的热门光催化材料。
但是它具有缺陷多、比表面积小和光生载流子易复合等缺点,光催化CO2还原性能不高.为此,介绍了高CO2还原活性的g-C3N4研究进展,内容包括:(1)g-C3N4研究基础(分子结构、制备方法与电子能带结构);(2)高活性g-C3N4的分子设计策略(缺陷调控、元素掺杂、表面等离子体处理、单原子催化和异质结构建等),重点讨论了改性方式对g-C3N4的光吸收、光电性能和CO2还原产物选择性的影响.最后建议未来聚焦结晶氮化碳的修饰改性研究,强调利用原位和瞬态表征技术指导高CO2还原活性的g-C3N4的开发,并关注具有高能量密度的长链碳氢燃料产物的选择性.关键词氮化碳;光催化;CO2还原;选择性中图分类号O625.67;O643.3 文献标志码 A 文章编号1672-4321(2023)06-0721-12doi:10.20056/ki.ZNMDZK.20230601Research progress of photocatalytic CO2 reduction ongraphitic carbon nitrideCHANG Shixin1,YU Mengxue1,YU Dai2,YAN Yi1*,WANG Zhi1,LYU Kangle1(1 College of Resources and Environment & Key Laboratory of Resources Conversion and PollutionControl of the State Ethnic Affairs Commission, South-Central Minzu University, Wuhan 430074, China;2 Hangzhou Inspection Institute of Quality and Technical Supervision, Hangzhou 310019, China)Abstract Semiconductor photocatalysis can use solar energy to drive the photocatalytic reduction of CO2,producing hydrocarbon fuel,which becomes a research hotspot. Graphitic carbon nitride (g-C3N4)is a popular photocatalytic material for CO2reduction,which has the merits of facile synthesis and visible-light-response property. However,the photocatalytic activity of g-C3N4 is not high enough for CO2 reduction due to its drawbacks including many defects, small specific surface area, and easy recombination of photogenerated charge carriers. Herein, the recent progress of high active g-C3N4 for CO2 reduction was introduced, which included (1) the research fundamental of g-C3N4: molecular structure,synthesis method,and electronic band structures;(2)the strategies of g-C3N4 molecular design for high efficient CO2 reduction:defects engineering,elements doping,surface plasma treatment,single-atom catalysis,and heterojunction construction. Detailed discussions were focused on theeffects of different modification methods on light absorption,photoelectric property,and selectivity of CO2reduction of g-C3N4. Finally,it is suggested to focus on the study of crystalline g-C3N4modification in the future,emphasizing the use of in situ and transient characterization techniques in exploration of g-C3N4with high CO2reduction activity and selectivity of long-chain hydrocarbon fuel products with high energy density.Keywords carbon nitride; photocatalysis; CO2 reduction; selectivity收稿日期2023-04-12* 通信作者严翼(1986-),女,讲师,博士,研究方向:环境生态,E-mail:****************基金项目国家自然科学基金资助项目(41901235)第 42 卷中南民族大学学报(自然科学版)工业革命以来,人类活动不断的增加和工业的迅速发展促使了化石燃料的大量使用,导致CO2温室气体的大量排放[1-4].伴随国家的“双碳”目标和绿色发展战略的提出,如何合理解决CO2气体造成的环境问题将影响社会和经济的可持续发展. CO2是一种比较稳定的分子,使C=O键断裂需要大约750 kJ‧mol-1的能量,常规的物理化学方法处理CO2较困难.但是分子中的O周围存在孤对电子,可以为路易斯酸中心提供电子,而其中C可以接受来自路易斯碱中心的电子[5];此外,CO2可以吸附在绝大多数催化剂材料表面上,这为催化还原CO2分子提供可能性[5-6].受光激发的半导体材料可以诱导CO2转化为高价值的碳氢燃料产物,在缓解温室效应的同时,还生产了高附加值工业化学品.因此,CO2的光催化还原具有节能和环保的优点,符合可持续发展的理念[7-8].随着研究的不断深入,高活性CO2还原的半导体光催化材料的开发也从初始的TiO2逐渐拓展到硫化物、金属氧化物和非金属氮碳化物等[9-10],这些催化剂的光吸收范围从紫外光逐渐向可见光拓展,CO2还原产物日渐丰富,从C1产物(如CO、CH4、CH3OH和HCOOH)过渡到C2产物(如C2H5OH 等)[5-6].在这些半导体材料中,氮化碳由于具有较好的物理化学稳定性、优异的光响应范围、合适的带隙结构、便捷的制备方式和易于改性等优点而受到广泛关注[3-4, 7].同时,由于氮化碳的能带结构满足光催化CO2还原的热力学条件,被迅速应用于CO2还原领域.但是,体相氮化碳仍然存在可见光吸收范围窄、载流子复合率高和比表面积小等缺点.针对这些问题,近年来研究人员致力于对氮化碳进行改性从而提升其光催化性能,特别是CO2还原产物的选择性,以产生更高价值的多碳产物.基于以上研究结果,本文主要针对氮化碳改性调节CO2还原产物的选择性进行总结,分别从缺陷调控、元素掺杂和构建异质结三个角度进行详细阐述,重点探讨了改性方法对于氮化碳光吸收、光电特性及还原产物选择性的影响,最后对氮化碳光催化材料未来发展提出展望.1 氮化碳的结构和性质氮化碳是一种热门的聚合型材料,拥有着较高的化学稳定性和热稳定性,耐酸碱腐蚀,最高可在700 ℃下保持热稳定性[4, 11].氮化碳前驱体在高温环境中,可以一步一步缩合成环状结构,这种环状结构的雏形最早由BERZELIUS发现,并在1834年由LIEBIG命名为“melon”[11-14].这种雏形材料继续进行缩合最终可得到两种氮化碳的主要结构——三嗪环(C3N3)[图1(a)]和七嗪环(C6N7)[图1(b)].这两种聚合型的结构由于缩合不完全,使少量杂质氢在结构边缘上产生伯胺基团或者仲胺基团,产生大量无序的体相缺陷.这些体相缺陷的存在,不利于光生载流子的快速迁移扩散,而成为了载流子复合中心,抑制光催化活性.所以,需要对氮化碳进行结构修饰与改性,提升其光催化性能[11, 15-16].氮化碳是一种典型的N型半导体材料,其能带结构如图1(c)所示,带隙约为2.7 eV,它的导带电位比大多数的CO2还原产物的电位更负,理论上可以生成诸多的还原产物.但在实际应用过程中,受到热力学和动力学因素的限制,氮化碳光催化CO2还原产物主要为CO和CH4[8].在CO2还原反应过程中,氮化碳价带上的空穴分解H2O为导带产物的生成提供H+[16];而导带上的电子还原CO2时,生成CH4比生成相同量的CO需要更多的电子和H+[公式(1)和公式(2)],所以生成CH4受到动力学因素的影响程度更大.此外,氮化碳材料的导带电位也满足生成H2的条件,这也制约了氮化碳还原CO2生成CH4[16-17].CO2 + 2H++ 2e-→ CO + H2OE0redox=-0.53 V (vs. NHE,PH = 7),(1)CO2 + 8H++ 8e-→ CH4+ 2H2OE0redox=- 0.21 V (vs. NHE,PH = 7).(2)氮化碳可以通过尿素、氰胺、双氰胺、三聚氰胺、硫脲等前驱体[图1(d)]通过热聚合(包括水热合成法、模板法、熔融盐法等)得到,方法便捷、易于批量制备[12-13, 18-20].其中,氰胺热缩合生成双氰胺,再由双氰胺热缩合生成三聚氰胺,最后通过三聚氰胺的逐渐缩合制备出氮化碳,这种途径被公认为是产生相对较少缺陷的聚合物的一种高效方法[4, 11].但是制备出的氮化碳存在较多缺陷,为了改善氮化碳缺陷多和载流子易复合的问题以提高光催化剂的活性和调节产物的选择性,可以从制备方式出发,通过缺陷调控、元素掺杂或修饰改性、构造异质结等途径实现氮化碳的高效应用和产物选择性的调控[21-23].对氮化碳进行改性处理后的CO2还原产物及选择性的结果详见表1.722第 6 期常世鑫,等:石墨相氮化碳光催化还原CO 2研究进展2 改性氮化碳调控CO 2光催化还原选择性CO 2的光催化还原,要经历多电子逐步还原的反应过程.CO 2在氮化碳表面的光催化还原产物主要有C 1产物和C 2产物,而生成更长链的多碳产物至今仍然面临着很大的挑战[5].C 1产物的生成过程, 首先是H +与电子转移到CO 2表面,生成羧基中间体(COOH*),然后进一步生成CO 、CH 4等产物[6].CO 由C =O*或C ≡O*生成,而其他C 1还原产物如HCHO 、CH 3OH 和CH 4的生成途径则由中间体CO*经过一系列反应生成[5].其中CH 4的生成方式有两种:一种通过CO*加氢生成CH 3O*,再转化成CH 4和H 2O ;另一种由CO*生成COH*,然后脱水形成C*,最后逐步加氢生成CH 4[5-6, 50].C 2产物由生成的CO*加氢生成*CHO ,然后碳碳键偶联产生COCHO*,继而生成乙醇和乙醛等产物[5, 24, 39].改性后的氮化碳因为性能发生改变会导致CO 2还原过程中热力学性能和动力学性能发生改变,使得生成的中间体的种类和相应的生成速率发生变化,最终影响到产物的选择性[5, 16].基于氮化碳的改性方式进行分类,本文将从多种氮化碳的改性方法对于产物选择性影响角度进行详细阐述.2.1 缺陷调控由于石墨相氮化碳的热聚合不完全,导致大量无序体相缺陷的生成,这些缺陷很容易成为光生载流子的复合中心,抑制石墨相氮化碳的光催化活性.但是,对于结晶度比较好在石墨相氮化碳,可以通过特定缺陷(如碳缺陷位点和氮缺陷位点)的引入来调控其半导体能带结构和表面化学环境,增强光吸收和载流子分离效率,实现CO 2还原的活性的增强和产物选择性的调控[1-2].氮空位的引入可以增强CO 2的吸附性能,同时可以作为陷阱诱捕光生电子,通过延长载流子的寿命和抑制载流子复合,来提升石墨相氮化碳的光催化还原CO 2性能[17].此外,捕获电子后的氮空位由于周围电子分布的改变更有利于CO 2吸附和活化[17].通过制备出的三聚氰胺-三聚氰酸超分子进行自组装制备出氮化碳(表1序号1),将氮化碳置于550 ℃下,使用氩气和氢气的混合气体氛围进行氢热处理制备出有氮空位缺陷的管状氮化碳[17].通过原位红外测试[图2(a )]可知:在反应图1 氮化碳结构、性质和制备方法Fig.1 Structure , properties and preparation method of carbon nitride723第 42 卷中南民族大学学报(自然科学版)表1 氮化碳改性策略与光催化还原CO2性能Tab.1 Modification strategies and photocatalytic CO2 reduction performances of carbon nitride序号1 234 5 6 7 8 9 10 11 12 13 14 15 16 17 18 19 20 21 22 23 24 25 26 27 28 29 30 31 32 33催化剂名称TCN-1NVs-PCNg-CN-650g-CN-750HCN-AP-g-C3N4S-CN1% B/g-C3N42Au-CNCN/Aug-C3N4/Bi/CDsNi25/g-CNCN/PDA10Def-CNPt@Def-CNCo-MOF/g-C3N4Ni5-CNCu-CCNCCNBsK-CNMn1Co1/CNPtCu-crCNInCu/PCNPd1+NPs/C3N4P/Cu SAs@CNC3N4/rGO/NiAl-LDHsCo3O4/CNSg-C3N4/Cu2Og-C3N4/Ti3C2TxCN/ZnO/GAg-C3N4/FeWO4PN-g-C3N4CeCo-PTI改性方法氮空位氮空位氮空位氮空位局部结晶P改性S掺杂B掺杂纳米Au纳米Au纳米Bi纳米Ni2,6吡啶二羧酸掺杂缺陷氮化碳单原子PtCo-MOFNi单原子Cu单原子Cu修饰K掺杂双单原子双单原子双单原子单原子与纳米粒子金属单原子与非金属Ⅱ型半导体Z型异质结Z型异质结异质结Z型异质结Z型异质结多孔纳米带S型异质结光源300 W氙灯LED灯300 W氙灯300 W氙灯LED灯300 W氙灯300 W氙灯300 W氙灯300 W氙灯氙灯300 W氙灯300 W氙灯500 W氙灯300 W氙灯300 W氙灯300 W氙灯300 W氙灯氙灯300 W氙灯氙灯300 W氙灯300 W氙灯300 W氙灯250 mW cm-2氙灯300 W氙灯300 W氙灯300 W氙灯300 W氙灯300 W氙灯300 W氙灯300 W氙灯300 W氙灯300 W氙灯活性/(µmol‧g-1‧h-1)CO: 7.1CO: 55.95CO: 5.3CH4: 34.4CH4: 52.8CH3COH: 1815CO: 2.4CH4: 1.8CO: 3.20CO: 0.45CH4: 0.16CH4: 1.55CO: 28.3CO: 9.08―CO: 284.7H2: 71CH4: 2.1CH4: 6.3CO: 6.75CH4: 5.47CO: 8.6CO: 3.1CO: 9.9H2: 0.94CO: 8.7CO: 47CH4: 2.8C2H5OH: 28.5CH4: 20.3C2H6: 616.6CO: 2.6CH4: 20.0CO: 13.3CH4: 3.2CH3OH: 0.71CO: 3.98CH4: 2.1CO: 33.9CO: 6.0CO: 29.8CH4: 45.4选择性100% CO85% CO86.6% CH496.4% CH498.3% CH3COH43.3% CH4100% CO26.2% CH419.1% CH495.6% CO98% CO28% CH4100% CO78% CH499% CH444.8% CH481.1% CO100% CO91.4% CO―100% CO19.4% CH492% C2H5OH97.8% CH433.0% C2H688.5% CH480.8% CO94.9% CH3OH34.7% CH492% CO91% CO100% CO88.3% CH4参考文献[17][22][16][16][24][25][26][27][28][29][30][31][32][33][33][3][34][35][36][23][37][38][39][40][41][42][43][44][45][46][47][48][49]724第 6 期常世鑫,等:石墨相氮化碳光催化还原CO 2研究进展过程中的产生了大量生成CO 的中间体——CH 3O*、HCOO -和COOH*,并未发现大量生成CH 4中间体,使CO 2还原更倾向于生成CO.除了改变煅烧热处理的气体氛围可以制造氮空位,利用甲酸辅助刻蚀也可以产生氮空位缺陷.杨朋举教授课题组[22]用三聚氰胺作为前驱体煅烧出氮化碳,利用氩气将甲酸带入管式炉对氮化碳进行热处理从而产生氮空位.通过表征发现氮空位主要集中在氮化碳的表面,形成氮空位后也极大地提高了CO 的产率,CO 选择性大约在85%(表1序号2).通过吉布斯自由能的理论计算[图2(b )]可以发现:这种方式引入的氮空位降低了生成COOH*的活化能,使得产物中CO 的选择性更高.氮空位的形成会影响材料能带结构,带隙的位置可以受到氮空位的电子密度的影响[16, 51].张金龙教授课题组[16]报道三聚氰胺在空气氛围下通过改变温度进行高温煅烧可以制备出氮空位的氮化碳.根据CO 2还原的活性测试结果发现:随着催化剂煅烧温度的提高,CH 4的选择性大幅提高,750 ℃煅烧出来的氮化碳CH 4的选择性最大并且达到96.4%(表1序号4).通过能带分析发现:随着煅烧温度的提高,间隙态的生成位置逐渐降低[图2(c )],在650 ℃以上的温度进行煅烧,间隙态的位置会低于产生CO 的电位.间隙态的产生会使得电子在激发后率先集中在其附近,更有利于从热力学方面产生CH 4.此外,利用Pt 4+在催化剂表面光沉积来研究氮化碳的光生电子的迁移途径发现:光生电子倾向于迁移并聚集在催化剂的边缘,导致边缘的氮缺陷处的电子密度更高,在动力学上对产生CH 4更有利.在热力学和动力学双重优势下,还原产物体现出更高的CH 4选择性.用传统方式热缩合得到的氮化碳基本为非晶态或半晶态的状态.在结晶氮化碳表面引入缺陷也是一种提升性能的方法.文献[24]通过加入氨基-2-丙醇(AP )和双氰胺制备氮化碳提高了单体的结晶度和聚合物的聚合度,获得了结晶氮化碳(图3).从样品的高分辨率透射电镜[图3(b )]照片,可以观察到明显的缺陷区域和有序的晶格条纹,反映出其缺陷氮化碳较高的结晶性能.这种结构可以促进CO 2向油类化合物的转化,通过对反应过程分析[图3(c )]可知:这种结构使得CO 2逐步生成C 2产物的中间体——CO*和CHO*,CO*和CHO*更容易自发偶联生成C 2产物中间体OCCHO*,抑制CHO*进行质子化的过程,故最终生成产物以CH 3CHO 为主,并且选择性高达98.3%(表1序号5).从理论上讲,相比将CO 2还原生成C 1产物,还原生成C 2产物具有更高的能量密度和更大的商业价值[52].2.2 元素掺杂元素掺杂改性也是一种常用的改性手段.金属或者非金属掺杂剂的原子轨道与催化剂本身的分图2 N 缺陷氮化碳CO 2还原选择性影响机理图Fig.2 Schematic diagram of selectivity reduction of CO 2 over carbon nitride with N defect725第 42 卷中南民族大学学报(自然科学版)子轨道发生杂化,能够起到改变反应的活性位点、调节能带结构和电子分布结构等作用,进而通过影响催化剂的性能来改变产物的选择性[5-6].在氮化碳还原CO 2过程中,电子从氮原子上激发并向碳原子上迁移,但是光激发后电子更加倾向于分布在氮附近,尤其是分布在双配位氮的附近[图4(a )],这使电子的迁移更加困难,导致在催化过程中载流子复合率高和反应动力更低[16, 27].刘敏教授课题组[27]建立了硼掺杂氮化碳的模型,根据模型[图4(b )]可知:硼原子已成功掺杂在相邻的七嗪环之间,并且与七嗪环的氮原子形成了良好的亲和力.通过计算发现在硼掺杂氮化碳后,激发后的电子从N (2P x ,2P y )向B (2P x ,2P y )上转移更加容易,可以极大地增加反应的动力,更有利于CH 4的产生.他们用硼酸和尿素混合进行一步煅烧实验生成硼掺杂氮化碳,硼作为主要的活性位点可以改变对CO 2还原中间体的吸附,使得产物更容易生成CH 4,所以相比纯氮化碳,生成CH 4的选择性得到了提高.相比硼掺杂,硫掺杂对氮化碳的性能改变有着不同影响.文献[26]通过水热和程序升温的方法制备出介孔硫掺杂氮化碳,更多的介孔形成和硫的掺杂增大了比表面积,并增强了对CO 2的吸附能力 [图5(a )],这有利于CO 2的活化并进行还原反应.在能带结构中,由于硫参与轨道杂化并且作为主要的活性位点,载流子的分离效率得到提高,反应的活性也得到增强.在生成产物的过程中,相比纯氮化碳,硫掺杂改性的氮化碳使生成的CO 产物更容易脱附[图5(b )],因此生成CO 的选择性显著提高.2.3 表面等离子体效应金属纳米粒子的负载可以增强光吸收和促进载流子分离,从而提高光催化活性的效果.在氮化碳上掺入金属纳米粒子后,不仅可以作为活性位点和形成促进载流子分离的肖特基结构显著提升性能,而且还会由于金属纳米粒子的局部表面等离子共振效应(LSPR )进一步拓展催化剂的光吸收范围[7, 53-54].负载Au 纳米粒子的氮化碳就是一个不错的例子,可以通过LSPR 效应一定程度上提高CH 4的选择性.KAIMIN S 教授课题组[28]利用NaBH 4还原法所制备的负载Au 纳米粒子的氮化碳,不仅有效地抑制了载流子复合,还通过LSPR 效应促进了更多热电子产生和增强了在可见光范围下的光吸收能力,大幅提高了CO 2还原的活性,尤其是为CH 4的形成提供了更多活性电子促进其生成.向全军教授课题组[29]用N 2等离子体处理浸渍在HAuCl 4中的氮化碳制备催化剂,这种Au 纳米粒子负载氮化碳也能通过Au 纳米粒子的LSPR 效应显著提高CH 4的选择性.图3 局部结晶氮化碳的结构与CO 2还原反应机理Fig.3 Morphology of locally crystalline carbon nitride and CO 2reduction reaction mechanism图4 B 掺杂氮化碳DFT 计算Fig.4 DFT calculation about B doped carbon nitride726第 6 期常世鑫,等:石墨相氮化碳光催化还原CO 2研究进展此外,金属纳米粒子作为活性位点也可以降低反应能垒.董帆教授课题组[30]使用碳点(CDs )作为基质,将Bi 纳米粒子锚定在氮化碳上并与其进行桥接制备出CNB -2,Bi 通过LSPR 效应增强了氮化碳光吸收的能力和产生了更多热电子,热电子产生后可自发注入氮化碳中,为CO 2还原提供更多热电子[图6(b )];而作为基质的CDs 可以作为光生空穴的受体,在内建电场的作用下Bi 和氮化碳所产生的空穴可以转移到CDs 上,有利于光生电荷的分离并为CO 2还原提供更多的还原动力.通过吉布斯自由能可以得出,Bi 纳米粒子的掺入明显降低生成CO 途径的中间产物的活化能,为生成CO 提供更多热力学条件,最终生成CO 的选择性得到了提高.2.4 单原子催化将金属由纳米级尺寸制备成更小的单原子尺寸,会引起原子自身特性发生更为显著的改变.通过金属单原子对氮化碳改性,一方面暴露出更多的单原子位点,影响吸附中心和反应位点;另一方面单原子通过改变电子结构对反应过程进行调整,拥有了更加出色的催化性能表现[54-56].金属单原子改性是一种充满挑战又极大提高催化剂性能的方法,有不少有关通过单金属单原子对氮化碳改性提升性能的报道.熊宇杰教授课题组[33]通过在氮化碳上分别负载Pt 单原子(Pt@Def -CN )和Pt 纳米粒子,进行CO 2还原实验中,相比未负载金属的氮化碳,它们的反应活性和CH 4的选择性显著提高,其中Pt@Def -CN 对于CH 4的选择性提升更高,达到了99%(表1序号15),由于单原子独特的性质对选择性造成了影响.一方面,因为H 原子与Pt 单原子之间结合相对不稳定,Pt 单原子附近存在更多—OH 基团,抑制了H 2产生,为生成CH 4提供更多H +;另一方面,Pt 单原子有效地降低了反应过程中生成CH 4的活化能能垒[图7(a )],同时又增加了CO*中间产物的解析能,提高了CH 4的选择性.向全军教授课题组[35]制备出掺入Cu 单原子的高结晶氮化碳,Cu 单原子的加入可作为CO 2活化的活性中心,提高了对CO 2的吸附能力,增强了反应活性.此外,Cu单原子的加入使图5 S 掺杂氮化碳的CO 2吸附等温线和CO -TPD 光谱Fig.5 CO 2 adsorption isotherms and CO -TPD spectra of S -oping carbon nitride图6 金属纳米离子改性氮化碳CO 2还原反应机理图Fig.6 Scheme diagram of metal nanoions modified carbon nitride CO 2 reduction reaction727第 42 卷中南民族大学学报(自然科学版)得生成CO 的反应过程优先于生成CH 4的反应过程[图7(b )],极大地提高了CO 的选择性.双金属单原子通过协同作用能提高CO 2还原性能.李亚栋教授课题组[37]合成出含有Co 和Mn 双金属单原子的氮化碳来进行CO 2还原.在还原过程中,光生空穴更倾向于移动到Mn 单原子上作为活性位点加速H 2O 分解,提供H +;而光生电子更倾向于移动到Co 单原子上,通过增加CO 2的键长和键角将CO 2活化,最终生成CO.这种双金属单原子的协同作用使CO 的选择性基本上达到100%(表1 序号21).侯军刚教授课题组[39]将Cu 和In 单原子分散在氮化碳上,双金属单原子的引入改变了催化剂的电子结构[图7(c )].在Cu 单原子附近有明显的电荷富集的迹象,而在In 单原子附近有明显的电荷消耗的迹象,它们之间的协同作用促进了电荷转移和电荷分离.此外,双金属的作用增强了对中间体CO*的吸附并降低了C —C 偶联的活化能,促使了偶联生成乙醇.金属单原子和金属纳米粒子同时引入氮化碳上能够协同发挥作用,调整CO 2还原的选择性.郑旭升教授课题组[40]通过在氮化碳上引入Pd 单金属(Pd 1)和Pd 纳米粒子(Pd NPs )作为双活性位点,改善了氮化碳的光催化性能.相比只引入Pd 1,双金属单原子引入后的协同作用使得CH 4的选择性有了显著提高[图7(d )].Pd NPs 的加入促进H 2O 分解并且加快H +转移到Pd 1;而Pd 1则更有利于吸附中间体CO*,加快质子化过程,生成CH 4.此外,Pd NPs 和Pd 1的协同作用也降低了从CO*到生成CHO*的活化能能垒,显著提高了生成CH 4的选择性.金属单原子与非金属之间也能够产生协同作用,提高氮化碳的性能,影响产物的选择性.毛俊杰教授[41]课题组报道了通过将P 和Cu 作为双活性位点锚定在氮化碳上,在CO 2还原过程中生成高选择性的C 2H 6产物.首先,P 和Cu 修饰对氮化碳的带隙起到一定调整作用,在一定程度上更有利于电子空穴的光激发分离.其次,P 和Cu 作为电子和空穴的捕获位点,可以促进Cu 对电子的富集从而实现CO 2还原的多电子过程.最后,P 和Cu 的修饰降低了中间生成C 2H 6的反应途径的活化能,CO*和CO*更容易发生偶联,形成中间体OCCO*,逐步加H最终生图7 单原子金属改性氮化碳CO 2还原反应机理图Fig.7 CO 2 reduction reaction scheme diagram of monometallic metal modified carbon nitride728第 6 期常世鑫,等:石墨相氮化碳光催化还原CO 2研究进展成C 2H 6产物.2.5 异质结构建不同于单一的材料,将复合材料制成异质结更有利于提高催化剂的性能.由于异质结界面在空间结构上彼此分离,光生电子和空穴的复合会更容易被抑制,从而改变生成产物的选择性[7, 21, 57].Ⅱ型异质结在CO 2还原的相关文献中经常被报道,汪铁林教授课题组[42]在NiAl 层状双金属氢氧化物(NiAl -LDHs )和氮化碳中引入还原氧化石墨烯(rGO )辅助制备成Ⅱ型半导体,由于rGO 拥有优异的导电子能力,能进一步促进载流子分离,使氮化碳上光生电子更迅速分离并转移到NiAl -LDHs 的Ni 原子上,导致生成CO 的选择性大大提高.Ⅱ型异质结虽然可以极大地促进载流子分离,但会使催化剂的价带或导带的电位降低[12, 21].Z 型异质结概念受到植物光合作用的机理启发提出.相比Ⅱ型异质结,Z 型异质结保持了更正的价带和更负的导带电位,因此复合材料拥有更强的光催化氧化/还原性能[21, 57-58],常应用于光催化领域.文献[46]报道利用静电自组装和低温共沉积法将ZnO 和氮化碳锚定在石墨烯气凝胶上制备出间接接触Z 型异质结结构,这种异质结结构的构建不仅使电子空穴更有效地空间分离,在CO 2还原产物中CO 的选择性更高.有国外课题组[47]制备出氮化碳和FeWO 4复合的直接接触Z 型异质结.同样地,这种异质结也极大地抑制了载流子分离和提高了氧化电位,使产物中H +更倾向于生成H 2,抑制了CH 4的产生,故CO 2还原的产物中没有CH 4和其他烃类产物产生.3 总结与展望光催化技术可以利用太阳能来驱动温室气体CO 2的催化还原,制备具有高附加值的碳氢燃料,因此该技术具有节能和环保的优点.在所有的半导体光催化材料中,石墨相氮化碳因为具有可见光响应和能带结构合理等优点,而成为受欢迎的CO 2还原光催化材料.但是,其依然存在缺陷多、比表面积小和光生载流子易复合等缺点,在一定程度上制约了该技术的实际应用.因此,科学家们采用各种策略对石墨相氮化碳进行修饰改性,以进一步提升其光催化还原CO 2的性能.本文总结了目前石墨相氮化碳用于CO 2还原方面的5种改性方式,分别是缺陷调控、元素掺杂、等离子体效应、单原子修饰和异质结构建.对石墨相氮化碳的结构修饰,改变了催化剂表面的化学环境,进而对CO 2光催化还原路径产生和产物还原选择性产生深远影响.为了实现CO 2在氮化碳表面的高效光催化还原,在今后的研究中以下工作值得进一步深入研究.(1)开展基于结晶石墨相氮化碳的修饰改性研究.相对于普通氮化碳,结晶氮化碳的体内和表面缺陷大幅度减少,而表现出高效载流子分离效率和光催化性能.但是石墨相氮化碳依然属于有机半导体材料,其表面缺乏过渡金属作为CO 2分子的吸附和活化中心.因此,需要开展基于结晶氮化碳的表面改性特别是过渡金属表面修饰研究.(2)开展修饰组分之间的协同作用机制研究.从CO 2在石墨相氮化碳表面的吸附开始,到吸附产物如CH 4/CO 的脱附,中间需要经历很多关键步骤.因此,深入研究各修饰组分之间的接力还原CO 2机制,对深刻理解CO 2还原的活性中心结构和指导高效光催化还原CO 2材料的开发具有重要意义.(3)开展CO 2光催化还原的原位瞬态谱学研究.CO 2分子在光催化还原过程中,存在中间产物结构图8 氮化碳异质结CO 2还原选择性机理图Fig.8 CO 2 reduction reaction scheme diagram of carbon nitride with heterojunction729。
【写作押题 中考英语】热点03 碳达峰碳中和(解析版)
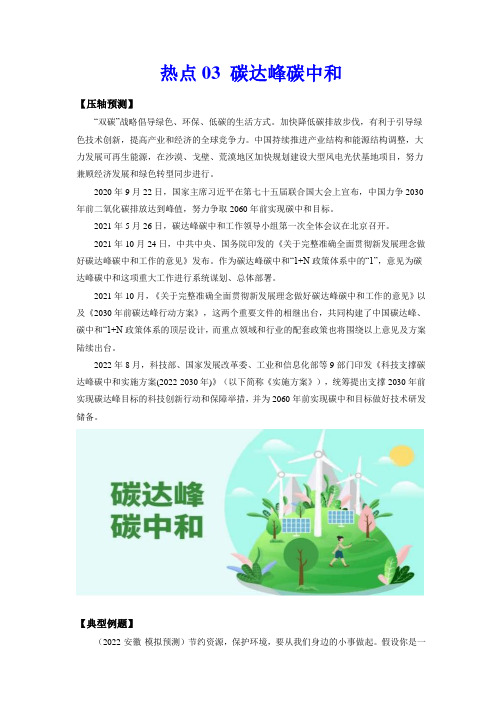
热点03 碳达峰碳中和【压轴预测】“双碳”战略倡导绿色、环保、低碳的生活方式。
加快降低碳排放步伐,有利于引导绿色技术创新,提高产业和经济的全球竞争力。
中国持续推进产业结构和能源结构调整,大力发展可再生能源,在沙漠、戈壁、荒漠地区加快规划建设大型风电光伏基地项目,努力兼顾经济发展和绿色转型同步进行。
2020年9月22日,国家主席习近平在第七十五届联合国大会上宣布,中国力争2030年前二氧化碳排放达到峰值,努力争取2060年前实现碳中和目标。
2021年5月26日,碳达峰碳中和工作领导小组第一次全体会议在北京召开。
2021年10月24日,中共中央、国务院印发的《关于完整准确全面贯彻新发展理念做好碳达峰碳中和工作的意见》发布。
作为碳达峰碳中和“1+N政策体系中的“1”,意见为碳达峰碳中和这项重大工作进行系统谋划、总体部署。
2021年10月,《关于完整准确全面贯彻新发展理念做好碳达峰碳中和工作的意见》以及《2030年前碳达峰行动方案》,这两个重要文件的相继出台,共同构建了中国碳达峰、碳中和“1+N政策体系的顶层设计,而重点领域和行业的配套政策也将围绕以上意见及方案陆续出台。
2022年8月,科技部、国家发展改革委、工业和信息化部等9部门印发《科技支撑碳达峰碳中和实施方案(2022-2030年)》(以下简称《实施方案》),统筹提出支撑2030年前实现碳达峰目标的科技创新行动和保障举措,并为2060年前实现碳中和目标做好技术研发储备。
【典型例题】(2022·安徽·模拟预测)节约资源,保护环境,要从我们身边的小事做起。
假设你是一个注重“低碳生活”的中学生,请以“My Low-carbon Life”为题写一篇英文演讲稿,简单谈谈“低碳生活”的重要性,以及你所倡导的“低碳生活”方式。
提示:1. 每天步行上学;2. 离开教室时,关闭灯、风扇电脑等;3. 不用水时,记住关水龙头,而且要循环使用水;4. 不使用塑料袋。
肟_肟醚_酯_类化合物的构型转化及其机理
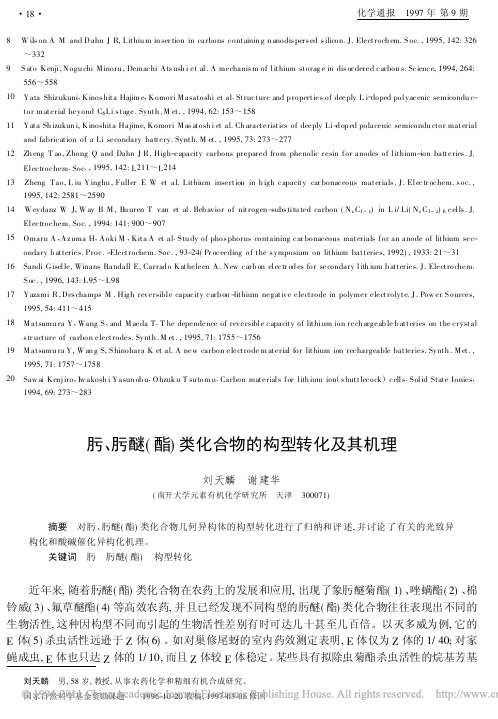
8 W ils on A M and Dahn J R,Lithiu m in ser tion in carbons containin g n anodis pers ed s ilicon.J.Electroch em.S oc.,1995,142:326~3329 S ato Kenji,Nogu chi M inoru,Demachi Ats ush i et al.A mechanis m of lithium storag e in dis or dered carbon s.Science,1994,264: 556~55810 Yata Shiz ukuni,Kinos hita Hajim e,Komori M asatoshi et al.Structure and p roperties of deeply L i-doped polyacenic semicondu c-tor m aterial beyond C6Li s tage.Synth.M et.,1994,62:153~15811 Yata Sh izukun i,Kinoshita Hajime,Komori M as atosh i et al.Ch aracteristics of deeply Li-d op ed polacenic semicondu ctor material and fabrication of a Li secondary battery.Synth.M et.,1995,73:273~27712 Zh eng T ao,Zhong Q and Dahn J R,High-capacity carbons prepared from phenolic resin for anodes of lithium-ion batteries.J.Electrochem.Soc.,1995,142:L211~L21413 Zheng Tao,L iu Yinghu,Fuller E W et al.Lithium insertion in h igh capacity car bonaceous materials.J.Electr ochem.s oc., 1995,142:2581~259014 W eydanz W J,W ay B M,Bu uren T van et al.Beh avior of nitrogen-subs titu ted carbon(N z C1-z)in L i/Li(N z C1-z)6cells.J.Electrochem.Soc.,1994:141:900~90715 Omaru A,Az uma H,Aoki M,Kita A et al.S tu dy of phos phorus containing car bonaceous materials for an anode of lithium sec-ondary b atteries.Proc.-Electrochem.Soc.,93-24(Pr oceeding of the s ymposium on lithium batteries,1992),1933:21~3116 Sandi Giselle,Winans Randall E,Carrad o Katheleen A.New carb on electr od es for secondary lith ium b atteries.J.Electrochem.S oc.,1996,143:L95~L9817 Yazami R,Des champs M.High reversible capacity carb on-lithium negative electrode in polymer electrolyte.J.Pow er S ources, 1995,54:411~41518 M atsumu ra Y,Wang S,and M aeda T.T he dependence of reversible capacity of lithium ion rech argeab le b atteries on the crystal s tr ucture of carbon electrodes.Synth.M et.,1995,71:1755~175619 M atsumu ra Y,W an g S,S hinohara K et al.A new carbon electrode m aterial for lithium ion rechargeable batteries.Synth.M et., 1995,71:1757~175820 Saw ai Kenjiro,Iw akosh i Yasun ob u,Ohzuk u T su tom u.Carbon materials for lith ium ion(s huttlecock)cells.S olid State Ionics, 1994,69:273~283肟、肟醚(酯)类化合物的构型转化及其机理刘天麟 谢建华(南开大学元素有机化学研究所 天津 300071)摘要 对肟、肟醚(酯)类化合物几何异构体的构型转化进行了归纳和评述,并讨论了有关的光致异构化和酸碱催化异构化机理。
阴极等离子体电解氧化表面改性新方法探索
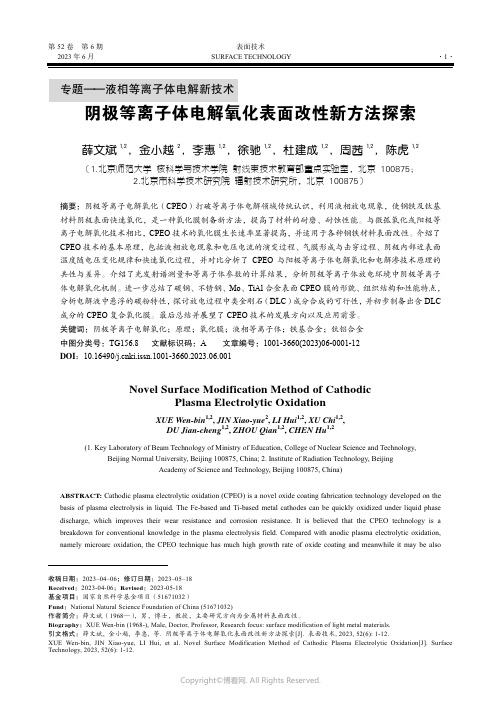
第52卷第6期表面技术2023年6月SURFACE TECHNOLOGY·1·专题——液相等离子体电解新技术阴极等离子体电解氧化表面改性新方法探索薛文斌1,2,金小越2,李惠1,2,徐驰1,2,杜建成1,2,周茜1,2,陈虎1,2(1.北京师范大学 核科学与技术学院 射线束技术教育部重点实验室,北京 100875;2.北京市科学技术研究院 辐射技术研究所,北京 100875)摘要:阴极等离子电解氧化(CPEO)打破等离子体电解领域传统认识,利用液相放电现象,使钢铁及钛基材料阴极表面快速氧化,是一种氧化膜制备新方法,提高了材料的耐磨、耐蚀性能。
与微弧氧化或阳极等离子电解氧化技术相比,CPEO技术的氧化膜生长速率显著提高,并适用于各种钢铁材料表面改性。
介绍了CPEO技术的基本原理,包括液相放电现象和电压电流的演变过程、气膜形成与击穿过程、阴极内部近表面温度随电压变化规律和快速氧化过程,并对比分析了CPEO与阳极等离子体电解氧化和电解渗技术原理的共性与差异。
介绍了光发射谱测量和等离子体参数的计算结果,分析阴极等离子体放电环境中阴极等离子体电解氧化机制。
进一步总结了碳钢、不锈钢、Mo、TiAl合金表面CPEO膜的形貌、组织结构和性能特点,分析电解液中悬浮的碳粉特性,探讨放电过程中类金刚石(DLC)成分合成的可行性,并初步制备出含DLC 成分的CPEO复合氧化膜。
最后总结并展望了CPEO技术的发展方向以及应用前景。
关键词:阴极等离子电解氧化;原理;氧化膜;液相等离子体;铁基合金;钛铝合金中图分类号:TG156.8 文献标识码:A 文章编号:1001-3660(2023)06-0001-12DOI:10.16490/ki.issn.1001-3660.2023.06.001Novel Surface Modification Method of CathodicPlasma Electrolytic OxidationXUE Wen-bin1,2, JIN Xiao-yue2, LI Hui1,2, XU Chi1,2,DU Jian-cheng1,2, ZHOU Qian1,2, CHEN Hu1,2(1. Key Laboratory of Beam Technology of Ministry of Education, College of Nuclear Science and Technology,Beijing Normal University, Beijing 100875, China; 2. Institute of Radiation Technology, BeijingAcademy of Science and Technology, Beijing 100875, China)ABSTRACT: Cathodic plasma electrolytic oxidation (CPEO) is a novel oxide coating fabrication technology developed on the basis of plasma electrolysis in liquid. The Fe-based and Ti-based metal cathodes can be quickly oxidized under liquid phase discharge, which improves their wear resistance and corrosion resistance. It is believed that the CPEO technology is a breakdown for conventional knowledge in the plasma electrolysis field. Compared with anodic plasma electrolytic oxidation, namely microarc oxidation, the CPEO technique has much high growth rate of oxide coating and meanwhile it may be also收稿日期:2023–04–06;修订日期:2023–05–18Received:2023-04-06;Revised:2023-05-18基金项目:国家自然科学基金项目(51671032)Fund:National Natural Science Foundation of China (51671032)作者简介:薛文斌(1968—),男,博士,教授,主要研究方向为金属材料表面改性。
碳纤维表面改性研究进展

Keywords: carbon fiber ;coating method ;plasma treatment ;surface grafting method ;surface oxidation method
美标材料对照表

COYARD SAS - FRANCETableau des alliages de fonderieASTM NORM GRADE UNS N°Designation Equivalent barre Densité (g/cm3)Fonderie COYARDAl Bi C Cr Cu Fe Mn Mo N Ni Nb P Pb Si SSn W VTensile strength,ksi (MPa)Yield strength,min ksi (MPa)in.(50mm),min,%Reduction of area, min, %Aluminium Bismuth Carbone Chrome CuivreFer Manganèse Molybdène Azote Nickel Niobium Phosphore Plomb Silicium Soufre Etain Tungstène VanadiumCarbon Chromium CopperIronManganese Molybdenum NitrogenNickelColumbium Phosphorus SiliconSulfur VanadiumCARBON STEELA216WCA J02502On request 0,25max 0,50max 0,30max Bal.0,70max 0,20max 0,50max 0,04max 0,60max 0,045max 0,03max 60 to 85 (415 to 585)30 (205)2435A216WCB J03002A105Standard 0,30max 0,50max 0,30max Bal.1,00max 0,20max 0,50max 0,04max 0,60max 0,045max 0,03max 70 to 95 (485 to 655)36 (250)2235A216WCC J02503Standard 0,25max 0,50max 0,30max Bal.1,20max 0,20max 0,50max 0,04max 0,60max 0,045max 0,03max 70 to 95 (485 to 655)40 (275)2235A217WC1J12524Standard 0,25/Bal.0,5-0,80,45-0,65//0,040,60,04565 to 90 (450 to 620)35 (240)2435A217WC4On request 0,05-0,200,50-0,80Bal.0,5-0,80,45-0,650,70-1,10/0,040,60,04570 to 95 (485 to 655)40 (275)2035A217WC5Standard 0,05-0,200,50-0,90Bal.0,4-0,70,90-1,200,60-1,00/0,040,60,04570 to 95 (485 to 655)40 (275)2035A217WC6J12072A182 F11Standard 0,05-0,201,00-1,50Bal.0,5-0,80,45-0,65//0,040,60,04570 to 95 (485 to 655)40 (275)2035A217WC9A182 F22On request 0,05-0,182,00-2,75Bal.0,4-0,70,90-1,20//0,040,60,04570 to 95 (485 to 655)40 (275)2035A217WC11On request 0,15-0,211,00-1,50Bal.0,5-0,80,45-0,65//0,0200,30-0,600,01580 to 105 (550 to 725)50 (345 )1845A217C5J42045Standard 0,204,00-6,50Bal.0,40-0,700,45-0,65//0,040,750,04590 to 115 (620 to 795)60 (415)1835A217C12J82090On request 0,208,00-10,00Bal.0,35-0,650,90-1,20//0,041,000,04590 to 115 (620 to 795)60 (415)1835A217C12A A182 F91On request 0,128,0-9,5Bal.0,30-0,600,85-1,050,400,060-0,100,0200,20-0,500,01885 to 110 (585 to 760)60 (415)2045A217CA15J91150410Standard0,1511,5-14,0Bal.1,000,501,00/0,0401,500,04090 to 115 (620 to 795)65 (450)1830AUSTENITIC & AUSTENITIC-FERRITIC (DUPLEX)A351CF3, CF3A J92500304L Standard 0,0317,0-21,0/Bal.1,500,50/8,0-12,0/0,0402,000,040//70 (485), 77 (530)30 (205), 35 (240)35,0A351CF8, CF8A J92600304Standard 0,0818,0-21,0/Bal.1,500,50/8,0-11,0/0,0402,000,040//70 (485), 77 (530)30 (205), 35 (240)35,0A351CF3M, CF3MA J92800316L Standard 0,0317,0-21,0/Bal.1,502,0-3,0/9,0-13,0/0,0401,500,040//70 (485), 80 (550)30 (205), 37 (255)30,0A351CF8M J92900316Standard 0,0818,0-21,0/Bal.1,502,0-3,0/9,0-12,0/0,0401,500,040//70 (485)30 (205)30,0A351CF3MN J92804316LN On request 0,0317,0-21,0/Bal.1,502,0-3,00,10-0,209,0-13,0/0,0401,500,040//75 (515)37 (255)35,0A351CF8C J92710347On request0,0818,0-21,0/Bal.1,500,50/9,0-12,0/0,0402,000,040//70 (485)30 (205)30,0A351CF10A182 F3210,04-0,1018,0-21,0/Bal.1,500,50/8,0-11,0/0,0402,000,040//70 (485)30 (205)35,0A351CF10M J929010,04-0,1018,0-21,0/Bal.1,502,0-3,0/9,0-12,0/0,0401,500,040//70 (485)30 (205)30,0A351CH80,0822,0-26,0/Bal.1,500,50/12,0-15,0/0,0401,500,040//65 (450)28 (195)30,0A351CH100,04-0,1022,0-26,0/Bal.1,500,50/12,0-15,0/0,0402,000,040//70 (485)30 (205)30,0A351CH200,04-0,2022,0-26,0/Bal.1,500,50/12,0-15,0/0,0402,000,040//70 (485)30 (205)30,0A351CK203100,04-0,2023,0-27,0/Bal.1,500,50/19,0-22,0/0,0401,750,040//65 (450)28 (195)30,0A351HK300,25-0,3523,0-27,0/Bal.1,500,50/19,0-22,0/0,0401,750,040//65 (450)35 (240)10,0A351HK400,35-0,4523,0-27,0/Bal.1,500,50/19,0-22,0/0,0401,750,040//62 (425)35 (240)10,0A351HT300,25-0,3513,0-17,0/Bal.2,000,50/33,0-37,0/0,0402,500,040//65 (450)28 (195)15,0A351CF10MC0,1015,0-18,0/Bal.1,501,75-2,25/13,0-16,0/0,0401,500,040//70 (485)30 (205)20,0A351CN7M N08007Alloy 20-UNS N08020-Carpenter 20CB-38,08Standard 0,0719,0-22,03,0-4,0Bal.1,502,0-3,0/27,5-30,5/0,0401,500,040//62 (425)25 (170)35,0A351CN3MN N08367AL6XNOn request0,03max 20,0-22,00,75max Bal.2,00max 6,0-7,00,18-0,2623,5-25,5/0,040max 1,00max 0,010max //80 (550)38 (260)35A351CD4MCu 0,0424,5-26,52,75-3,25Bal.1,001,75-2,25/4,75-6,00/0,041,000,04//100 (690)70 (485)16,0A351CE8MN 0,0822,5-25,5/Bal.1,003,0-4,50,10-0,308,0-11,0/0,0401,500,040//95 (655)65 (450)25,0A351CG6MMN 0,0620,50-23,50/Bal.4,00-6,001,50-3,000,20-0,4011,50-13,500,10-0,300,0401,000,030/0,10-0,3085 (585)42,5 (295)30,0A351CG8M J93000317Standard0,0818,0-21,0/Bal.1,503,0-4,0/9,0-13,0/0,041,500,04//75 (515)35 (240)25,0A351CF10SMnN 0,1016,0-18,0/Bal.7,00-9,00/0,08-0,188,0-9,0/0,0603,50-4,500,030//85 (585)42,5 (295)30,0A351CT15C 0,05-0,1519,0-21,0/Bal.0,15-1,50//31,0-34,00,50-1,500,030,50-1,500,03//63 (435)25 (170)20,0A351CK3MCuN S31254A182 F44 - 254-SMOOn request 0,02519,5-20,50,50-1,00Bal.1,206,0-7,00,18-0,2417,5-19,5/0,0451,000,010//80 (550)38 (260)35A351CE20N 0,2023,0-26,0/Bal.1,500,500,06-0,208,0-11,0/0,0401,500,040//80 (550)40 (275)30A351CG-3M J92999317L Standard 0,0318,0-21,0/Bal.1,503,0-4,0/9,0-13,0/0,041,500,04//75 (515)35 (240)25A351CD3MWCuN 0,0324,0-26,00,5-1,0Bal.1,003,0-4,00,20-0,306,5-8,5/0,0301,000,0250,5-1,0/100 (700)65 (450)25,0A351CN2MCuNN08904904L / Uranus B67,95Standard0,0219,0-23,01,0-2,0Bal.2,0max4,0-5,023,0-28,00,045max1,00max 0,035max65,0028,0035,00CARBON STEELA352LCA Carbon Steel On request 0,250500,300,700,200,500,040,600,0450,0360,0-85,0 (415-585)30,0 (205)24A352LCB J03003Carbon SteelASTM A352 LF2On request 0,300,500,30Bal.1,000,200,500,040,600,0450,0365,0-90,0 (415-585)35,0 (240)2435A352LCC J02505Carbon-Manganese Steel Standard 0,250,500,30Bal.1,200,200,500,040,600,0450,0370,0-95,0 (485-655)40,0 (275)2235A352LC1Carbon-Molybdenum SteelOn request 0,250,50-0,0800,45-0,650,040,600,04565,0-90,0 (450-620)35,0 (240)2435A352LC221/2% Nickel SteelOn request 0,250,50-0,802,00-3,000,040,600,04570,0-95,0 (485-655)40,0 (275)2435A352LC2-1Nickel-Chromium-Molybdenum SteelOn request 0,221,35-1,850,55-0,750,30-0,602,50-3,500,040,500,045105,0-130,0 (725-895)80,0 (550)1830A352LC331/2% Nickel Steel On request 0,150,50-0,803,00-4,000,040,600,04570,0-95,0 (485-655)40,0 (275)2435A352LC441/2% Nickel Steel On request 0,150,50-0,804,00-5,000,040,600,04570,0-95,0 (485-655)40,0 (275)2435A352LC99% Nickel SteelOn request 0,130,500,300,900,208,50-10,00,040,450,0450,0385,0 (585)75,0 (515)2030A352CA6NM121/2%Chromium, Nickel-Molybdenum SteelOn request0,0611,5-14,01,000,4-1,03,5-4,50,041,000,03110,0-135,0 (760-930)80,0 (550)1535NICKEL ALLOYSA494CZ-100N02100Cast Nickel 1,001,25max 3,00max 1,5095,00min 0,032,000,0350,00 (345)18,00 (125)10,00A494M-35-1N24135Monel 4008,8Standard 0,3526,0-33,03,50max 1,50Bal.0,50max 0,031,250,0365,00 (450)25,00 (170)25,00A494M-35-2N04020Monel On request0,3526,0-33,03,50max 1,50Bal.0,50max0,032,000,0365,00 (450)30,00 (205)25,00N05500Monel K5008,47A494M-30H 0,3027,0--33,03,50max 1,50Bal.0,032,7-3,70,03100,00 (690)60,00 (415)10,0A494M-25S On request0,2527,0-33,03,50max 1,50Bal.0,033,5-4,50,03A494M-30C N24130Weldable Monel 0,3026,0-33,03,50max1,50Bal.1,0-3,00,031,0-2,00,0365,00 (450)32,50 (225)25,00A494N-12MVN30012Hastelloy BOn request0,121,004,0-6,01,0026,0-30,0Bal.0,0401,000,0300,20-0,6076,00 (525)40,00 (275)6,00A494N-7M N30007Hastelloy B29,22On request 0,071,03,00max 1,0030,0-33,0Bal.0,0401,000,03076,00 (525)40,00 (275)20,00A494CY-40N06040Inconel 6008,47On request 0,4014,0-17,011,00max 1,50Bal.0,033,000,03070,00 (485)28,00 (195)30,00A494CW-12MW N30002Hastelloy C On request 0,1215,5-17,54,5-7,51,0016,0-18,0Bal.0,0401,000,0303,75-5,250,20-0,4072,00 (495)40,00 (275)4,00A494N10276Hastelloy C-2768,89Standard 0,0215,5-17,54,5-7,516,0-18,0Bal.3,75-5,250,20-0,4072,00 (495)40,00 (275)4,00A494CW-6M N30107Hastelloy C Modified On request 0,0717,0-20,03,0max 1,0017,0-20,0Bal.0,0401,000,03072,00 (495)40,00 (275)25,00A494CW-2M N26455Hastelloy C4C 0,0215,0-17,52,0max 1,0015,0-17,5Bal.0,030,800,031,0max72,00 (495)40,00 (275)20,00A494CW-6MC N26625Inconel 6258,44On request0,0620,0-23,05,0max 1,008,0-10,0Bal.3,15-4,500,0151,000,01570,00 (495)40,00 (275)25,00A494CY5SnBiM 3,0-5,00,0511,0-14,02,0max 1,52,0-3,5Bal.0,030,50,033,5-5,0A494CX2MW N26022Hastelloy C-228,690,0220,0-22,52,0-6,01,0012,5-14,5Bal.0,0250,800,0252,5-3,50,35max80,00 (550)45,00 (280)30,0A494Cu5MCuCN08825Incoloy 8258,140,050max 19,5-23,51,50-3,50Bal.1,0max2,5-3,538,0-44,00,60-1,200,030max1,0max 0,030max75,00 (520)35,00 (240)20,0N08800Incoloy 8007,94IRON-CHROMIUM-NICKEL-MOLYBDENUM & DUPLEX (AUSTENITIC/FERRITIC) ALLOYSA8901A (CD4MCu)J93370Duplex Grade 1A On request0,04max 24,5-26,52,75-3,25Bal.1,00max 1,75-2,254,75-6,000,040max 1,00max 0,04max /100 (690)70 (485)16A8902A (CE8MN)J93345Duplex Grade 2A On request 0,08max 22,5-25,5/Bal.1,00max 3,00-4,500,10-0,308,00-11,000,04max 1,50max 0,04max /95 (655)65 (450)25A8903A (CD6MN)J93371Duplex Grade 3AOn request 0,06max 24,0-27,0/Bal.1,00max 1,75-2,500,15-0,254,00-6,000,040max 1,00max 0,04max /95 (655)65 (450)25A8904A (CD3MN)J92205Duplex Grade 4A A182 F51 - AISI 22-05 - UNS S31803 7,8Standard 0,03max 21,0-23,51,00maxBal.1,50max 2,5-3,50,10-0,304,5-6,50,04max 1,00max 0,020max /90 (620)60 (415)25A8905A (CE3MN)J93404Duplex Grade 5A A182F53 - AISI 25-07 - UNS S32750On request 0,03max 24,0-26,0/Bal.1,50max 4,0-5,00,10-0,306,0-8,00,04max 1,00max 0,04max /100 (690)75 (515)18A8906A (CD3MWCuN)J93380Grade 6A Zeron100A182F55 - UNS S32760On request0,03max 24,0-26,00,5-1,0Bal.1,00max 3,0-4,00,20-0,306,5-8,50,030max 1,00max 0,025max 0,5-1,0100 (700)65 (450)25A8901B (CD4MCuN)J933720,04max 24,5-26,52,7-3,3Bal.1,00max 1,7-2,30,10-0,254,7-6,00,04max 1,00max 0,04max /100 (700)70 (485)16A8907AFerralium 255UNS S32550COPPER-NICKEL ALLOYSB369C9640070/30 Copper-Nickel On request 0,15Bal.0,25-1,51,5max 28,0-32,060,0032,0020ALUMINIUM-BRONZEB148Grade A (9A)C95200Aluminium Bronze On request 8,5-9,5Bal.2,5-4,065 (450)25 (170)20B148Grade B (9B)C95300Aluminium Bronze On request 9,00-11,0Bal.0,75-1,565 (450)25 (170)20B148Grade C (9C)C95400Aluminium Bronze On request 10,0-11,583,0min 3,0-5,00,50max 1,5max 75 (515)30 (205)12B148C95410Aluminium Bronze On request 10,0-11,5Bal.3,0-5,00,50max 1,5-2,575 (515)30 (205)12B148Grade D (9D)C95500Nickel Aluminium Bronze Standard 10,0-11,578,0min 3,0-5,03,5max 3,0-5,590 (620)40 (275)6B148Grade E (9E)C95600Silicon Aluminium BronzeOn request 6,0-8,0Bal.0,25max 1,75-3,2560 (415)28 (195)10B148Grade F (9F)C95700Manganese Nickel Aluminium BronzeOn request 7,0-8,5Bal.2,0-4,011,0-14,01,5-3,00,03max 0,10max 90 (620 )40 (275)20B148C95800Nickel Aluminium Bronze 7,64Standard 8,5-9,578,0min 3,5-4,50,8-1,54,0-5,00,03max 0,10max85 (585)35 (240)15B148C95900Aluminium BronzeOn request 12,0-13,5Bal.3,0-5,01,5max 0,5maxCaractéristiques mécaniquesComposition , %07/02/2006。
表面含氧官能团与铜负载对活性炭吸附甲硫醇的影响

第52卷第7期 辽 宁 化 工 Vol.52,No. 7 2023年7月 Liaoning Chemical Industry July,2023收稿日期: 2022-07-24表面含氧官能团与铜负载 对活性炭吸附甲硫醇的影响 喻阳,王俊卿,胡雪燕,郭江峰(中船动力(集团)有限公司,上海 200129)摘 要:甲硫醇是一种典型的恶臭污染物,嗅觉阈值非常低,其所引起的环境污染已经不容忽视。
吸附法工艺简单,成本低,适用范围广,并且改性活性炭对于甲硫醇气体表现出了优异的吸附性能。
采用硝酸氧化改性法提升活性炭表面含氧官能团含量,并且使用物理浸渍法在活性炭上负载铜离子。
探究了无氧条件下,含氧官能团以及铜负载对于活性炭吸附甲硫醇的影响,分析了含氧官能团以及铜负载两者的联合作用以及甲硫醇在活性炭上的吸附机理。
关 键 词:含氧官能团;活性炭;甲硫醇;吸附中图分类号:TQ424.1 文献标识码: A 文章编号: 1004-0935(2023)07-0967-04近几年,随着人们对于生活和生态环境的重视,对于恶臭污染的投诉越来越多[1]。
恶臭气体来源广泛,工业、养殖业、市政废物处理等行业都会产生恶臭气体[2-4]。
从垃圾填埋场和下水道中恶臭成分分析来看,除H2S外,其次便是甲硫醇,并且甲硫醇的嗅觉阈值极低[5-6]。
目前对于甲硫醇的处理方法主要分为催化法、微生物法、溶剂吸收法、吸附法,应用较多的是微生物法和吸附法[7]。
微生物法产物一般为CO2、H2O、硝酸盐以及硫酸盐的化合物,具有生态安全性[8-9]。
但是微生物法筛选菌群周期长,容易受到外界环境影响[10]。
吸附法工艺简单,处理效果、稳定性和经济性都非常显著。
活性炭的孔隙结构发达,在甲硫醇的吸附容量上已经表现出了优异的吸附性能[11]。
本文采用硝酸对活性炭进行氧化改性,并且联合物理浸渍法在不同改性活性炭上进行金属负载。
探究了无氧条件下,活性炭表面含氧官能团以及金属负载对于活性炭甲硫醇吸附性能的影响,对甲硫醇在改性活性炭上的吸附产物和机理进行了分析。
丙二酸二乙酯的合成进展
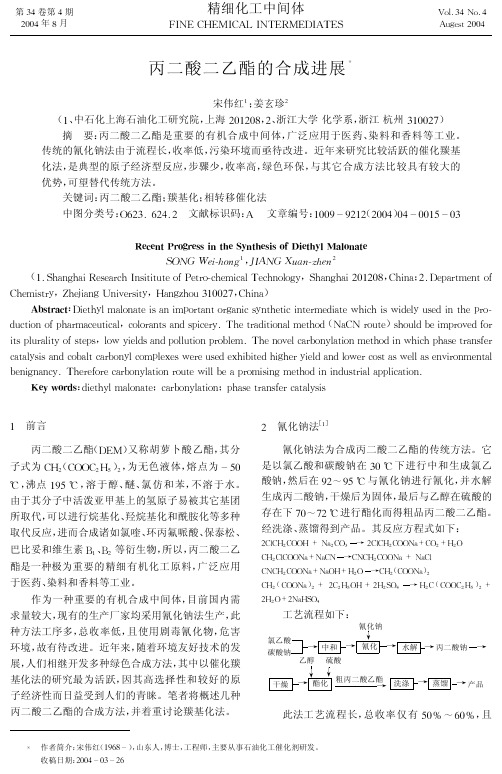
丙二酸二乙酯的合成进展!宋伟红1;姜玄珍2(1、中石化上海石油化工研究院,上海201208,2、浙江大学化学系,浙江杭州310027)摘要:丙二酸二乙酯是重要的有机合成中间体,广泛应用于医药、染料和香料等工业。
传统的氰化钠法由于流程长,收率低,污染环境而亟待改进。
近年来研究比较活跃的催化羰基化法,是典型的原子经济型反应,步骤少,收率高,绿色环保,与其它合成方法比较具有较大的优势,可望替代传统方法。
关键词:丙二酸二乙酯;羰基化;相转移催化法中图分类号:O 623.624.2文献标识码:A文章编号:1009-9212(2004)04-0015-03R ecent pro g ress i n t he S y nt hesis of d iet h y l m alonateSONG W ei -hon g 1,JI ANG XMOn -zhen2(1.S han g hai research I nsitit ute o f petro -che m ical T echno lo gy ,S han g hai 201208,Chi na ;2.D e p art m ent o fChe m istr y ,Zhe j ian g U ni versit y ,H an g Zhou 310027,Chi na )Abstract :D iet h y l m alonate is an i m p ortant or g anic s y nt hetic i nter m ediate Which is W i del y used i n t he p ro-duction o f p har m aceutical ,co lorants and s p icer y .T he traditional m et hod (N aCN route )should be i m p roved f or its p l uralit y o f ste p s ,loW y iel ds and p o llution p roble m.T he novel carbon y lation m et hod i n Which p hase transf er catal y sis and cobalt carbon y l com p lexes W ere used exhi bited hi g her y ield and loW er cost as W ell as environ m ental beni g nanc y .T heref ore carbon y lation route W ill be a p rom isi n g m et hod i n i ndustrial a pp lication.K e y words :diet h y l m alonate ;carbon y lation ;p hase transf er catal y sis1前言丙二酸二乙酯(DEM )又称胡萝卜酸乙酯,其分子式为CH 2(COOC 2H 5)2,为无色液体,熔点为-50C ,沸点195C ,溶于醇、醚、氯仿和苯,不溶于水。
富羧酸基团的共轭微孔聚合物:结构单元对孔隙和气体吸附性能的影响
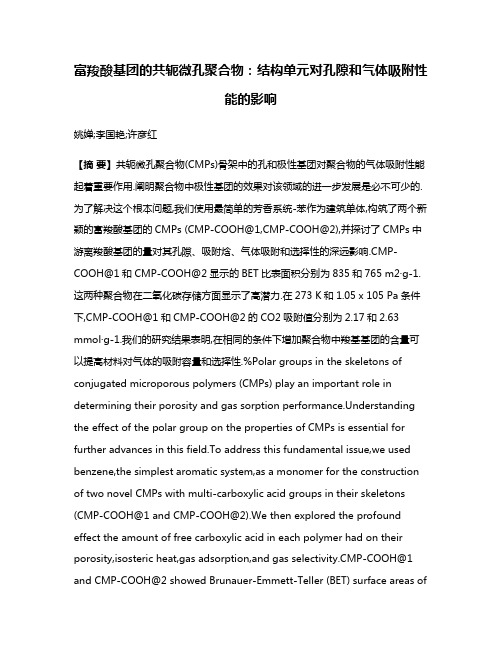
富羧酸基团的共轭微孔聚合物:结构单元对孔隙和气体吸附性能的影响姚婵;李国艳;许彦红【摘要】共轭微孔聚合物(CMPs)骨架中的孔和极性基团对聚合物的气体吸附性能起着重要作用.阐明聚合物中极性基团的效果对该领域的进一步发展是必不可少的.为了解决这个根本问题,我们使用最简单的芳香系统-苯作为建筑单体,构筑了两个新颖的富羧酸基团的CMPs (CMP-COOH@1,CMP-COOH@2),并探讨了CMPs中游离羧酸基团的量对其孔隙、吸附焓、气体吸附和选择性的深远影响.CMP-COOH@1和CMP-COOH@2显示的BET比表面积分别为835和765 m2·g-1.这两种聚合物在二氧化碳存储方面显示了高潜力.在273 K和1.05 x 105 Pa条件下,CMP-COOH@1和CMP-COOH@2的CO2吸附值分别为2.17和2.63 mmol·g-1.我们的研究结果表明,在相同的条件下增加聚合物中羧基基团的含量可以提高材料对气体的吸附容量和选择性.%Polar groups in the skeletons of conjugated microporous polymers (CMPs) play an important role in determining their porosity and gas sorption performance.Understanding the effect of the polar group on the properties of CMPs is essential for further advances in this field.To address this fundamental issue,we used benzene,the simplest aromatic system,as a monomer for the construction of two novel CMPs with multi-carboxylic acid groups in their skeletons (CMP-COOH@1 and CMP-COOH@2).We then explored the profound effect the amount of free carboxylic acid in each polymer had on their porosity,isosteric heat,gas adsorption,and gas selectivity.CMP-COOH@1 and CMP-COOH@2 showed Brunauer-Emmett-Teller (BET) surface areas of835 and 765 m2·g-1,respectively,displaying high potential for carbon dioxide storage applications.CMP-COOH@1 and CMP-COOH@2 exhibited CO2 capture capabilities of 2.17 and 2.63 mmol·g-1 (at 273 K and 1.05 x 105 Pa),respectively,which were higher than those of their counterpart polymers,CMP-1 and CMP-2,which showed CO2 capture capabilities of 1.66 and 2.28 mmol·g-1,respectively.Our results revealed that increasing the number of carboxylic acid groups in polymers could improve their adsorption capacity and selectivity.【期刊名称】《物理化学学报》【年(卷),期】2017(033)009【总页数】7页(P1898-1904)【关键词】共轭微孔聚合物;羧酸;孔;气体吸附;选择性【作者】姚婵;李国艳;许彦红【作者单位】吉林师范大学,环境友好材料制备和应用教育部重点实验室,长春130103;吉林师范大学,环境友好材料制备和应用教育部重点实验室,长春130103;吉林师范大学,环境友好材料制备和应用教育部重点实验室,长春130103;吉林师范大学,功能材料物理与化学教育部重点实验室,吉林四平136000【正文语种】中文【中图分类】O647Carbon dioxide is one of the main greenhouse gases that cause global issues, such as climate warming and increases in sea level and oceanacidity. Modern climate science predicts that the accumulation of greenhouse gases in the atmosphere will contribute to an increase ins urface air temperature of 5.2 °C between the years 1861 and 2100. Carbon capture and sequestration (CCS), a process of CO2 separation and concentration can contribute to solve. For this aim, the use of porous materials tailored for selective CO2 absorption is energetically efficient and technically feasible. Among the numerous and diversified examples of novel porous materials, such as metal-organic frameworks1,2, zeolites3,4, and purely organic materials5,6 are a class of porous organic materials that allow an elaborate design of molecular skeletons and a fine control of nanopores.Conjugated microporous polymers (CMPs) are a unique class of porous organic materials that combine π-conjugated skeletons with permanent nanopores7–10, which is rarely observed in other porous polymers. CMPs have emerged as a powerful platform for synthesizing functional materials that exhibit excellent functional applications, such as heterogeneous catalysts11,12, guest encapsulation13–15, super-capacitive energy storage devices16,17, light-emitting materials18,19, and fluorescent sensors20,21 and so on. Recently, CMPs have emerged as a designable material for the adsorption of gases, such as hydrogen, carbon dioxide, and methane22–24. Although great achievements in synthesizing CMPs have been realized, extremely high Brunauer-Emmet-Teller specific surface areas as high as 6461 m2·g−125, the other pore parameters, such as pore volume, pore size, and pore size distribution, are important in determining the gas sorptionperformance26,27. Moreover, previous work has shown the surface modification of porous polymers with polar group can significantly enhance their CO2 binding energy, resulting in enhancement in CO2 uptake and/or CO2 selectivity28–30. Carboxylic-rich framework interaction is expected due to hydrogen bonding and/or dipole-quadrupole interactions between CO2 and the functional groups of porous polymers31,32. Cooper et al.33,34 reported increasing the heat of adsorption through the introduction of tailored binding functionalities could have more potential to increase the amount of gas adsorbed. Their results demonstrated that carboxylic groups functionalised polymer showed the higher isosteric heat of sorption for CO2. Torrisi et al.35 predicted that the incorporation of carboxylic groups would lead to the higher isosteric heat, challenging the current research emphasis in the literature regarding amine groups for CO2 capture.Herein, we report the synthesis and characterization two high carboxylic groups of porous polymers and investigate their performances in CO2 storage application under high pressure and cryogenic conditions (Scheme 1, CMP-COOH@1 and CMP-COOH@2). The CMPs are highly efficient in the uptake of CO2 by virtue of a synergistic structural effect, and that the carboxylic units improve the uptake, the high porosity provides a large interface, and the swellable skeleton boosts the capacity.1,3,5-Triethynylbenzene (98%) was purchased from TCI, 2,5-dibromobenzoic-3-carboxylic acid (97%) and 2,5-dibromoterephthalicacid(97%) were purchased from Alfa. Tetrakis(4-ethynylphenyl)methane was synthesized according to the literature36. Tetrakis(triphenylphosphine)palladium(0), copper(I) iodide (CuI) andtetra(4-bromophenyl)methane (97%) were purchased from Aladdin. N,N-Dimethylformamide (DMF) (99.9%), triethylamine (99%), methanol (95%) and acetone (95%) were purchased from Aladdin.1H NMR spectra were recorded on Bruker Avance III models HD400 NMR spectrometers, where chemical shifts (δ) were determined with a residual proton of the solventas standard.Fourier transform Infrared (FT-IR) spectra were recorded on a Perkin-Elmer spectrum one model FT-IR-frontier infrared spectrometer.The UV-visible analyzer was used for shimadzu UV-3600. Field-emission scanning electron microscopy (FE-SEM) images were performed on a JEOL model JSM-6700 operating at an accelerating voltage of 5.0 kV. The samples were prepared by drop-casting a tetrahydrofunan (THF) suspension onto mica substrate and then coated with gold.High-resolution transmission electron microscopy (HR-TEM) images were obtained on a JEOL model JEM-3200 microscopy.Powder X-ray diffraction (PXRD) data were recorded on a Rigaku model RINT Ultima III diffractometer by depositing powder on glass substrate, from 2θ = 1.5° up to 2θ = 60° with 0.02° increment. The elemental analysis was carried out on a EuroEA-3000. TGA analysis was carried out using a Q5000IR analyzer with an automated vertical overhead thermobalance. Before measurement, the samples were heated at a rate of 5 °C min−1 under a nitrogen atmosphere. Nitrogen sorption isotherms were measured at 77 K with ASIQ (iQ-2) volumetric adsorption analyzer.Before measurement, thesamples were degassed in vacuum at 150 °C for 12 h. The Brunauer-Emmett-Teller (BET) method was utilized to calculate the specific surface areas and pore volume. BET surface areas were calculated over the relative pressure (p/p0) range of 0.015–0.1. Nitrogen NLDFT pore size distributions were calculated from the nitrogen adsorption branch using a cylindrical pore size model. Carbon dioxide, methane and nitrogen sorption isothermswere measured at 298 or 273 K with a Bel Japan Inc. model BELSORP-max analyzer, respectively. In addition, carbon dioxide sorption isotherms were measured at 318 K and 5 × 106 Pa with a iSorb HP2 analyzer. Before measurement, the samples were also degassed in vacuum at 120 °C for more than 10 h.2.2.1 Synthesis of CMP containing carboxylic groupsAll of the polymer networks containing multi-carboxylic groups were synthesized by palladium(0)-catalyzed cross-coupling polycondensation. All the reactions were carried out at a fixed reaction temperature and reaction time (120 °C/48 h).2.2.2 Synthesis of CMP-COOH@1 and CMP-COOH@22,5-Dibromoterephthalic acid (107 mg, 0.33 mmol) and 1,3,5-triethynylbenzene (50 mg, 0.33 mmol) (CMP-COOH@1)/tetrakis(4-ethynylphenyl)methane (104 mg, 0.25 mmol) (CMP-COOH@2) were put into a 50 mL round-bottom flask, the flask exchanged three cycles under vacuum/N2. Then added to 2 mL N,N-dimethylformamide (DMF) and 2 mL triethylamine (Et3N), the flask was degassed by threefreeze-pump-thaw cycles, purged with N2. When the solution had reached reactiontemperature, a slurry of tetrakis(triphenylphosphine)palladium(0) (23.11 mg, 0.02 mmol) in the 1 mL DMF and copper(I) iodide (4.8 mg, 0.025 mmol) in the 1 mL Et3N (CMP-COOH@1)/(CMP-COOH@2) was added respectively, and the reaction was stirred at 120 °C under nitrogen for 48 h. The solid product was collected by filtration and washed well with hot reaction solvent for 4 times with THF, methanol, acetone, and water, respectively. Further purification of the polymer was carried out by Soxhlet extraction with methanol, and THF for 24 h, respectively, to give CMP-COOH@1(claybank solid, 98 mg, 94% yield), CMP-COOH@2(olivine solid, 142 mg, 90% yield). Elemental Analysis (%) Calcd. (Actual value for an infinite 2D polymer), (CMP-COOH@1) C 67.61, H 2.35. Found: C 64.84, H 2.05. (CMP-COOH@2) C 73.03, H 3.02. Found: C 70.02, H 2.19. Carboxylic-CMP was synthesized by the Sonogashira- Higihara reaction of 1,3,5-triethynylbenzene, tetrakis(4- ethynylphenyl)methane and 2,5-dibromoterephthalic acid in the presence of Pd(0) as catalyst. These two samples were unambiguously characterized by elemental analysis confirmed that the weight percentages of C and H contents are close to the calculated values expected for an infinite 2D polymer. The CMPs were further characterized by infrared spectroscopy (Fig.1). Band soft he primary bromo and borate groups of 2,5-dibromoterephthalic acid at about 598 and 1368 cm−1are absent, respectively. From 2900 to 3200 cm−1aromatic C―H stretching bands appear. A C=C stretching mode at 1600 cm−1is also observed. All networks show the typical C≡C and COOH stretching mode at about 2200and 1700 cm−1, respectivel y, indicating the successfulincorporation of the carboxylic and alkynyl groups into the polymer materials.Field-emission scanning electron microscopy (FE-SEM) displayed that the CMPs adopt a spherical shape with sizes of 100–500 nm (Fig.2). High-resolution transmission electron microscopy (HR-TEM) revealed the homogeneous distribution of nanometer-scale pores in the textures (Fig.S1 (Supporting Information)). Powder X-ray diffraction (PXRD) revealed no diffraction, implying that all the polymers are amorphous (Fig.S2 (Supporting Information)). The TGA results show that the polymers have a good thermal stability, and the thermal degradation temperature is up to ca. 300 °C (Fig.S3 (Supporting Information)). The weight loss below 100 °C is generally attributed to the evaporation of adsorbed water and gas molecules trapped in the micropores.The conjugated polymer networks were dispersed in THF to obtain UV/Vis spectra (Fig.S4 (Supporting Information)). The polymer CMP-COOH@1 shows mainly one wide absorption peak at about 396 nm. Compared to monomer 1,3,5-triethynylbenzene, with narrow absorption maxima at 305 nm, the polymer networks exhibit a large bathochromic shift of around 111 nm. CMP-COOH@2 show similar phenomenon, compared totetrakis(4-ethynylphenyl)methane monomer, with absorption maxima at 325 and 345 nm, the polymer frameworks display a large bathochromic shift of around 68 and 48 nm, respectively. This indicates the effective enlargement of the π-conjugated system through the polycondensation reaction.The porosity of the polymer networks was probed by nitrogen sorption at 77 K. According to the IUPAC classification37, adsorption/desorption isotherms of two polymers showed mainly a type I isotherms. As seen in Fig.3(a), remarkably, the two polymer samples exhibit a steep uptake at a relative pressure of p/p0 < 0.1, suggesting that these samples have micropores. There is a sharp rise in the isotherm for the CMP-COOH@1 at higher relative pressures (p/p0 > 0.8), which indicates the presence of meso/macropores in the samples. These textural meso/macropores can be also found in the corresponding FE-SEM images (Fig.2(a)). However, the shape of the isotherm for the CMP-COOH@2 is significantly different from that of CMP-COOH@1, which displays a significant H2 type hysteresis loop in the desorption branch, characteristic of nanostructured materials with a mesoporous structure (Fig.3(a)). These meso/macropores can be ascribed mostly to interparticulate porosity that exists between the highly aggregated nanoparticles38.The pore size distribution calculated from nonlinear density functional theory (NLDFT) shows that the two polymer networks have relatively broad pore size distribution (Fig.3(b)). CMP-COOH@1 and CMP-COOH@2 showed apparent peaks in the size range 0–2 nm, whereas small fluctuations can be observed at 2–12 nm regions. The pore size distribution curves agree with the shape of the N2 isotherms (Fig.3(a)) and imply the presence of both micropores and mesopores in the two polymers. The contribution of microporosity to the networks can be calculated as the ratio of the micropore volume (Vmicro), over the totalpore volume (Vtotal). The microporosities of CMP-COOH@1 and CMP-*******************%and52.3%,respectively.Thisresultindicates that the two carboxylic networks are predominantly microporous. In addition, the BET surface area of CMP-COOH@1 and CMP-COOH@2 were calculated to be 835 and 765 m2·g−1 in the relative pressure range 0.015–0.1, respectively. The decreased surface area of CMP-COOH@2 compared to CMP-COOH@1 could be due to the CMPs constructed with longer connecting struts have lower BET surface areas39,40.In view of the fact that the CMPs possess two key properties generally associated with high CO2 uptake capacity, e.g., good porosity and abundant COOH sites, the CO2 adsorption of the polymers were investigated up to 1.05 × 105 Pa at both 298 K and 273 K (Fig.4(a, b)), respectively. Remarkably, CMP-COOH@1 and CMP-COOH@2 showed the CO2 adsorption capacities of 1.61 and 1.92 mmol·g−1 at 298 K and 1.05 × 105 Pa, respectively (Fig.4(a)). When the temperature was elevated to 273 K, the polymers CMP-COOH@1 and CMP-COOH@2 displayed the higher CO2 capture of 2.17 and 2.63 mmol·g−1(Fig.4(b)), respectively, which were comparable to that of other microporous hydrocarbon networks41. Despite CMP-COOH@2 with a lower surface area, but which adsorbed more CO2 probably due to it has a higher pore volume. In addition, the isosteric heat of adsorption (Qst) of the polymers was calculated from the CO2 uptake data at 273 K and 298 K by using Clausius-Clapeyron equation (Fig.4(c)). The two polymer networks show the isosteric heats of CO2 adsorption around 35.5 and 30.9 kJ·mol−1. Because there is less carboxylicacid in the structural unit, the CO2Qst of CMP-COOH@2 is lower than that of CMP-COOH@1, which is consistent with that of the previous reported polymers33,34. Moreover, the high pressure CO2 sorption properties of the two polymers were also investigated at 5 × 106 Pa and 318 K. As seen in Fig.4(d), CMP-COOH@1 and CMP-COOH@2 show a nearly linear increase with the increasing pressure no obviously turning point. CMP-COOH@1 and CMP-COOH@2 show the higher CO2 capture capacity of 498 and 434 mg·g−1 at 318 K and 5 × 106 Pa, respectively (Fig.4(d)). These results indicated that the CO2 uptake in these networks at high pressures is not dependent solely on the surface area, pore volume or polar groups in the skeletons, but also the measuring pressure have a large effect on the uptake of gas.In order to investigate the amount of carboxylic group in the network whether affects CO2 adsorption capacity of polymers. We synthesized another two carboxylic conjugated polymer with relatively low amount of carboxylic groups (scheme S1, CMP@1 and CMP@2 (Supporting Information)) based on 2,5-dibromobenzoic acid, 1,3,5-triethynylbenzene and tetrakis(4-ethynylphenyl)methane. They show the BET surface area of 979 and 876 m2·g−1 (Fig.S5 (Supporting Information)), respectively, which is higher to that of counterpart CMP-COOH@1 and CMP-COOH@2. CMP@***********************************–2.0 nm (Fig.S6 (Supporting Information)). The decreased surface area of CMP-COOH@1 compared to CMP@1 could be due to the volume of 2,5-dibromoterephthalic acid in CMP-COOH@1 is obviously larger than 2,5-dibromobenzoic acid in CMP@1, which made the bulky benzen–carboxylic **************************************************** phenomenon can be also observed in CMP-COOH@2 and CMP@2 system. As shown in Fig.4(b), at 273 K and 1.05 × 105 Pa, polymers C MP@1 and****************************************.28mmol·g−1, respectively. The CO2 uptake value of CMP-COOH@1 and CMP-COOH@2 is 1.31 and 1.15-times that of the counterpart CMP@1 and CMP@2, respectively, indicating that increasing amount of carboxylic groups in the CMP networks can improve CO2 uptake. In addition, we calculated the isosteric heats of these polymers, they showed the following order (Fig.4(c)):CMP-COOH@1>CMP-COOH@2>CMP@1>************* there is less carboxylic groups in the structural units of CMP@1 andCMP@2, the CO2Qst of CMP@1 and CMP@2 is lower than that of CMP-COOH@1 and CMP-COOH@2, respectively33,42. In addition, CMP-COOH@1 and CMP-COOH@2 show the higher CO2 capture capacity than that of CMP@1 (447 mg·g−1) and CMP@2 (402 mg·g−1) at 318 K and 5 × 106 Pa, respectively (Fig.4(d)). These results imply the amount of carboxylic groups effects BET surface area, pore volume and isosteric heats lead to different the uptake of gas.As for carbon dioxide capture, high separation properties towards CH4 and N2 are also necessary and important in gas separation applications. In order to investigate the gas adsorption selectivity of the microporous polymer networks, CO2, N2, and CH4 sorption properties were measured by volumetric metho ds at 273 K and 1.05 × 105 Pa. It was found that thetwo porous polymer networks show significantly higher CO2 uptake ability than N2 and CH4 in the whole measurement pressure range (Fig.S7 (Supporting Information)). CO2/CH4 and CO2/N2 selectivity was first evaluated by using the initial slope ratios estimated from Henry′s law constants for single-component adsorption isotherms. The CO2/CH4 selectivityofCMP-COOH@********************************** and 6.2, respectively (Table S1 and Fig.S8 (Supporting Information)). In addition, two polymers exhibited the CO2/N2 adsorption selectivity is 48.2 and 39.5, respectively (Table S1 and Fig.S9 (Supporting Information)). Meanwhile, the gas selective capture was also supported by the results from the ideal adsorbed solution theory (IAST), which has been widely used to predict gas mixture adsorption behavior in the porous materials43,44. Under simulated natural gas conditions (CO2/CH4, 50/50), the experimental CO2 and CH4 isotherms collected at 273 K for carboxylic CMP were fitted to the dual-site Langmuir model and the single-site Langmuir model, respectively (Fig.S10 (Supporting Information)). The calculated IAST data for carboxylic CMP are shown in Table S1. At 273 K and 1.05 × 105 Pa, CMP-COOH@1 and CMP-COOH@2 exhibit an appreciably high selectivity of CO2 over CH4 under natural gas conditions (5.5 and 5.2) (Fig.S10 (Supporting Information)), which is comparable to some reported MOPs, such as A6CMP (5.1) 45, SCMP (4.4–5.2) 30, and P-G1-T (5) 46. Furthermore, the CO2/N2 adsorption selectivities for CMP-COOH@****************************************.8at273K and 1.05 × 105 Pa (Table S1 and Fig.S11 (Supporting Information)),respectively, which is comparable to some reported MOPs, such as ALP-1(35) 38, PCN-TA (33) 47, and PCN-DC (48) 47. These excellent CO2 selective capture performance of carboxylic CMPs evaluated by IAST are consistent with the results calculated from the initial slopes method. In addition, in light of the amount of carboxylic group effect for the uptake of gas, we reasoned that it might be effective for CO2/CH4 and CO2/N2 separations. At 273 K and 1.05 × 105 Pa, CMP@1 and CMP@2 exhibit the selectivities of CO2/CH4 (4.7 and 4.1) and CO2/N2 (32.1 and 30.5) under natural gas conditions via the IAST method (Figs.S10 and S11 (Supporting Information)), respectively, which are lower that of counterpart CMP- COOH@************************************************* carboxylic groups effects selectivity of polymers. These data implys that increasing the amount of carboxylic unit of polymers can improve the adsorption capacity and selectivity of the materials, which suggested the possibility for the surface properties of microporous polymers to be controlled to interact with a specific gas by post-modification.In summary, two carboxylic CMPs with relatively high surface area have been synthesized. The clean energy applications of the polymers have also been investigated and it was found that CMP-COOH@1 and CMP-***********************.63mg·g−1 of carbon dioxide at 1.05 × 105 Pa and 273 K, respectively, which can be competitive with the reported results for porous organic polymers under the same conditions. The free carboxylicacid functionalized polymers show that increasing the amount of carboxylic group of polymers can improve the adsorption capacity andselectivity of the materials under the same conditions, which is a promising candidate for the separation and purification of CO2 from variousCO2/CH4 mixtures such as natural gas and land-fill gas by adsorptive processes.Supporting Information: available free of charge via the internet at.【相关文献】(1) Sumida, K.; Rogow, D. L.; Mason, J. A.; McDonald, T. M.; Bloch, E. D.; Herm, Z. R.; Baeand, T. H.; Long, J. R. Chem. Rev.2012,112, 724. doi: 10.1021/cr2003272(2) Suh, M. P.; Park, H. J.; Prasad, T. K.; Lim, D. W. Chem. Rev. 2012,112, 782. doi:10.1021/cr200274s(3) Coudert, F. X.; Kohen, D. Chem. Mater.2017,29, 2724. doi:10.1021/acs.chemmater.6b03837(4) Jensen, N. K.; Rufford, T. E.; Watson, G.; Zhang, D. K.; Chan, K. I.; May, E. F. J. Chem. Eng. Data2012, 57, 106. doi: 10.1021/je200817w(5) Tan, L.; Tan, B. Chem. Soc. Rev. 2017, doi: 10.1039/C6CS00851H(6) Ghanem, B.S.; Hashem, M.; Harris, K. D. M.; Msayib, K. J.; Xu, M.; Budd, P. M.; Chaukura, N.; Book, D.; Tedds, S.; Walton, A.; McKeown, N. B. Macromolecules2010,43, 5287. doi: 10.1021/ma100640m(7) Xu, Y. H.; Jin, S. B.; Xu, H.; Nagai, A.; Jiang, D. Chem. Soc. Rev.2013,42, 8012.doi:10.1039/C3CS60160A(8) Cooper, A. I. Adv. Mater.2009,21, 1291. doi: 10.1002/adma.200801971(9) Thomas, A.; Kuhn, P.; Weber, J.; Titirici, M. M.; Antonietti, M. Macromol. Rapid. Commun.2009,30, 221. doi: 10.1002/marc.200800642(10) Dawson, R.; Cooper, A. I.; Adams, D. J. Prog. Polym. Sci. 2012, 37, 530.doi:10.1016/j.progpolymsci.2011.09.002(11) Zhang, K.; Kopetzki, D.; Seeberger, P. H.; Antonietti, M.; Vilela, F. Angew. Chem. Int. Ed.2013, 52, 1432. doi: 10.1002/anie.201207163(12) Xie, Z.; Wang, C.; deKrafft, K. E.; Lin, W. J. Am. Chem. Soc.2011,133, 2056.doi:10.1021/ja109166b(13) Li, A.; Sun, H. X.; Tan, D. Z.; Fan, W. J.; Wen, S. H.; Qing, X. J.; Li, G. X.; Li, S. Y.; Deng,W. Q. Energy Environ. Sci.2011,4, 2062. doi: 10.1039/C1EE01092A(14) Wang, X. S.; Liu, J.; Bonefont, J. M.; Yuan, D. Q.; Thallapally, P. K.; Ma, S. Q. Chem. Commun.2013,49, 1533. doi: 10.1039/C2CC38067F(15) Bhunia, A.; Vasylyeva, V.; Janiak, C. Chem. Commun. 2013,49, 3961. doi:10.1039/C3CC41382A(16) Kou, Y.; Xu, Y.; Guo, Z.; Jiang, D. Angew. Chem. Int. Ed.2011,50, 8753. doi:10.1002/anie.201103493(17) Zhuang, X.; Zhang, F.; Wu, D.; Forler, N.; Liang, H.; Wagner, M.; Gehrig, D.; Hansen, M. R.; Laquai, F.; Feng, X. Angew. Chem. Int. Ed.2013,52, 9668. doi:10.1002/anie.201304496(18) Xu, Y.; Nagai, A.; Jiang, D. Chem. Commun.2013, 49, 1591. doi:10.1039/C2CC38211C(19) Xu, Y.; Chen, L.; Guo, Z.; Nagai, A.; Jiang, D. J. Am. Chem. Soc. 2011, 133, 17622. doi: 10.1021/ja208284t(20) Liu, X.; Xu, Y.; Jiang, D. J. Am. Chem. Soc.2012,134, 8738. doi: 10.1021/ja303448r(21) Gu, C.; Huang, N.; Gao, J.; Xu, F.; Xu, Y.; Jiang, D. Angew. Chem. Int. Ed.2014,53, 4850. doi: 10.1002/anie.201402141(22) Liao, Y.; Weber, J.; aul, C. F. J. Chem. Commun.2014, 50, 8002. doi:10.1039/C4CC03026E(23) Lu, W.; Sculley, J. P.; Yuan, D.; Krishna, R.; Wei, Z.; Zhou, H. C. Angew. Chem. Int. Ed. 2012,51, 7480. doi: 10.1002/anie.201202176(24) Xiang, Z.; Cao, D.; Wang, W.; Yang, W.; Han, B.; Lu, J. J. Phys. Chem. C2012,116, 5974. doi: 10.1021/jp300137e(25) Yuan, D.; Lu, W.; Zhan, D.; Zhou, H. Adv. Mater.2011,23, 3723. doi:10.1002/adma.201101759(26) Pu, L.; Sun, Y.; Zhang, Z. J. Phys. Chem. A2010,114, 10842. doi: 10.1021/jp103331a(27) Babarao, R.; Jiang, J. W. Langmuir2008,24, 6270. doi; 10.1021/la800369s(28) Islamoglu, T.; Rabbani, M. G.; El-Kaderi, H. M. J. Mater. Chem. A2013,1, 10259. doi: 10.1039/C3TA12305G(29) Hasmukh, A. P.; Ferdi, K.; Ali, C.; Joonho, P.; Erhan, D.; Yousung, J.; Mert, A.; Cafer, T. Y. J. Mater. Chem.2012,22, 8431. doi: 10.1039/c2jm30761h(30) Qin, L.; Xu, G.; Yao, C.; Xu, Y. Polym. Chem.2016,7, 4599. doi:10.1039/C6PY00666C(31) Rabbani, M. G.; El-Kaderi, H. Chem. Mater.2011,23, 1650. doi:10.1021/cm200411p(32) Arab, P.; Rabbani, M. G.; Sekizkardes, A. K.; İsllamoğlu, T.; El-Kaderi, H. M. Chem. Mater.2014,26, 1385. doi: 10.1021/cm403161e(33) Dawson, R.; Adams, D. J.; Cooper, A. I. Chem. Sci.2011,2, 1173. doi:10.1039/C1SC00100K(34) Dawson, R.; Cooper, A. I.; Adams, D. J. Polym. Int.2013,62, 345.doi:10.1002/pi.4407(35) Torrisi, A.; Mellot-Draznieks, C.; Bell, R. G. J. Chem. Phys.2010,132, 044705. doi: 10.1063/1.3276105(36) Li, P. Z.; Wang, X. J.; Liu, J.; Lim, J. S.; Zou, R.; Zhao, Y. J. Am. Chem. Soc.2016, 138, 2142. doi: 10.1021/jacs.5b13335(37) Rose, M.; Klein, N.; Bohlmann, W.; Bohringer, B.; Fichtner, S.; Kaskel, S. Soft Matter2010,6, 3918. doi: 10.1039/C003130E(38) Chen, Q.; Luo, M.; Hammershoj, P.; Zhou, D.; Han, Y.; Laursen, B. W.; Yan, C. G.; Han, B. H. J. Am. Soc. Chem. 2012,134, 6084. doi: 10.1021/ja300438w(39) Jiang, J. X.; Su, F. B.; Trewin, A.; Wood, C. D.; Niu, H. J.; Jones, J. T. A.; Khimyak, Y. Z.; Cooper, A. I. J. Am. Chem. Soc.2008,130, 7710.doi: 10.1021/ja8010176(40) Jiang, J. X.; Trewin, A.; Adams, D. J.; Cooper, A. I. Chem. Sci.2011,2, 1777. doi:10.1039/C1SC00329A(41) Meng, B.; Li, H.; Mahurin, S. M.; Liu, H.; Dai, S. Rsc. Adv.2016,6, 110307. doi:10.1039/C6RA18307G(42) Ma, H.; Ren, H.; Zou, X.; Meng, S.; Sun, F.; Zhu, G. Polym. Chem.2014,5, 144. doi; 10.1039/C3PY00647F(43) Obrien, J. A.; Myers, A. L. Ind. Eng. Chem. Res.1988, 27, 2085. doi:10.1039/C3PY00647F(44) Wang, K.; Qiao, S. Z.; Hu, X. J. Sep. Purif. Technol.2000,20, 243. doi:10.1016/S1383-5866(00)00087-3(45) Qin, L.; Xu, G.; Yao, C.; Xu, Y. Chem. Commun.2016,52, 12602. doi:10.1039/C6CC05097B(46) Qiao, S.; Wang, T.; Huang, W.; Jiang, J. X.; Du, Z.; Shieh, F.; Yang, R. Polym. Chem.2016,7, 1281. doi: 10.1039/C5PY01767J(47) Shen, C.; Yan, J.; Deng, G.; Zhang, B.; Wang, Z. Polym. Chem.2017,8, 1074. doi: 10.1039/C6PY02050J。
高考英语外刊时文精读专题03看到空中的碳足迹

高考英语外刊时文精读精练 (3)Carbon emissions碳排放Seeing footprints in the air看到空中的碳足迹主题语境:人与自然主题语境内容:环境保护【外刊原文】(斜体单词为超纲词汇,认识即可;下划线单词为课标词汇,需熟记。
)Chris Jones of the University of California, Berkeley, was on a river in the Amazon rainforest when he put the fin ishing touches on the world’s first online household carbon calculator(计算器). That was in 2005. He hoped that, if he could show people how much greenhouse gas was associated with daily activities—driving the car, heating the house—they might change their behaviour and contribute in some small measure to saving the Amazon. Seventeen years later, trackers are providing a wealth of often-neglect ed information about the carbon emissions of everyday life. They provide local and micro data which usefully supplement the global findings of the Intergovernmental Panel on Climate Change.Trackers work by asking users to answer questions such as: how many miles a year do you drive; how much is your annual household electricity bill; how often do you eat meat? They then calculate a personal or household estimate of emissions of carbon-dioxide equivalent (CO2e,二氧化碳当量排放量) per year. Alex Beale, a climate blogger in Atlanta who has studied them, reckons there are dozens of household carbon trackers and hundreds of specialist ones, including those which calculate emissions from food or other industries, such as a new one from the Stockholm Environment Institute (SEI) to track emissions from shipping. For individuals, reckons Mr Beale, the most comprehensive are the Cool Climate tracker run by Dr Jones at Berkeley and the calculator set up by the World Wildlife Fund (WWF) and SEI. What do they tell us?Dr Jones describes the main household polluting activities as “cars, coal, cows and consumption,roughly in that order”. By f ar the largest single source of emissions is the family vehicle. One car of average fuel efficiency driven 14,000 miles (22,500km) spews out 7 tonnes of carbon, according to Dr Jones’s tracker. Swapping it for an electric vehicle would save over 6 tonnes, or an eighth of the average American household’s yearly emissions.No other change would generate that much saving, though electricity in the home is responsible for over 5 tonnes of carbon emissions a year, so generating itwith solar panels(太阳能电池板) would come close . Like electric vehicles, a roof full of solar panels is not cheap. Changing diets costs less, and American households consume meat worth 2.7 tonnes of CO2e a year, far more than most people. If Americans went vegetarian(素食者), that would be like half an average solar roof.These household averages, however,disguise what may be the most important thing carbon trackers reveal: that apparently similar households produce very different emissions. By combining their tracker’s results with postal(邮政的)code data, the University of California team worked out average emissions by area. Places with high emissions—mostly suburbs(郊区)—produce four or five times as much carbon as inner cities or rural areas, a much larger multiple than might have been expecte d. Chicago’s households produce37 tonnes of CO2e a year; suburban Eola’s, some35 miles (56km) from the Windy City, emit96 tonnes. This is not only because of commuting(通勤). Trips to and from work account for less than a fifth of miles driven; the rest are to shops, schools and so on.Even more striking is the difference air travel makes. The average household contribution from flying is 1.5 tonnes, less than a car. But half of Americans never fly. According to Cool Climate, flying 100,000 miles a yearproduces a stunning(惊人的)43 extra tonnes of CO2. If jet-set households were to cut their travel sharply, they would have a disproportionate(不成比例的)effect on emissions. They might even do something for the Amazon.Over the next 30 years, many countries are promising to move to net-zero carbon, imply ing that household emissions will have to be cut to close to nothing. Stephanie Roe, WWF’s lead climate scientist, reckons that, at best, half the reduction might be achieved through demand-side measures, such as behavioural changes by individuals and households. And even that would require companies and governments to provide more incentives(激励)to change through supply-side investments to make low-carbon options cheaper and more widely available.Trackers, it seems, have daunting(令人怯步的)lessons for public bodies and private households alike.【课标词汇】1.associate将…(与…)联系起来,把…联系在一起Most people associate this brand with good quality.大多数人把这个品牌和优良品质联系在一起。
碳纤维表面化学镀镍对Cf_Al复合材料界面及耐蚀性的影响

M.H.Vidal-sc’tif,M.Lancin,C.Marhic,etal.Mater.&£.Engin.A.1999。272:32 1-333. 于志强,武高辉。孙东立.铝基复合材料增强体涂层与界面.材料工程.2001,10:13-17. Wielage
B,Dorner
A.Compos.&£Techn01.1999(59):1239-1245.
Wang
(School
Abstract
Chunyu,Jiang Longtao,Zhang
Qiang,Wu Gaohui
1 5000 1)
of Materials Science and Engineering,Harbin Institute of Technology,Harbin
The
surface modification of carbon fibers by electroless plating nickel is
2 2
SnCl2+HxO_Sn(OH忙I+旷十Cl
GeAr复合材料界面
使}}j镀镍和来镀镍f|勺碳纤维分别制作c,A1复
SnC|2+H徊叶sn(OHh+2H++2C|
反应生成的Sn(OHEI和sn(oH)-2结台形成微溶于水 的Sn2(OH)3CI吸附丁碳纤维表面。活化时,活化剂 被还原形成催化晶核,使以后的化学镀可以在这些袭 面上进行。活化处理是将敏化处理后的碳纤维浸入含 有催化活性的贵金属溶液中,进行再处理,使碳纤维 表面生成一层具有催化活性的贵金属层。活化原理: Pd2+十Sn2+一Sn舢+Pd 具有催化活性的金属微粒Pd就是化学镀镍的结品 核心。以上每一个前处理环节进行完斤都要认真清
得能有效传递载荷,调:i3.应力分布,阻f}:裂纹扩展
低碳钢液相等离子体电解B+C+N_共渗层的摩擦电化学行为

表面技术第52卷第6期低碳钢液相等离子体电解B+C+N共渗层的摩擦电化学行为王彬1,周茜2,3,高川力2,3,李惠2,3,金小越3,薛文斌2,3(1.山西农业大学 基础部,山西 太谷 030801;2.北京师范大学 核科学与技术学院射线束技术教育部重点实验室,北京 100875;3.北京市科学技术研究院 辐射技术研究所,北京 100875)摘要:目的研究液相等离子电解硼碳氮三元共渗处理(PEB/C/N)对Q235低碳钢摩擦电化学行为的影响。
方法采用PEB/C/N方法在Q235低碳钢表面制备共渗层,通过电化学的开路电位测试和摩擦磨损实验评估Q235钢基体和PEB/C/N试样在NaCl(质量分数3.5%)腐蚀介质中与Si3N4球对磨的摩擦电化学行为。
结果在电压为280 V的PEB/C/N共渗中,试样周围等离子体区的电子温度稳定在3 500 K左右。
经过PEB/C/N处理30 min后,生成的共渗层包括15 μm主要由Fe2B相组成的表面渗层和40 μm的过渡层。
在摩擦过程中,PEB/C/N试样的开路电位保持在−200~−300 mV之间,且波动较小,明显高于Q235钢基体。
同时,PEB/C/N试样的磨损率为3.88×104 μm3/(N m),只是钢基体磨损率的1/3。
在NaCl腐蚀介质中,由于腐蚀和磨损的交互作用,使Q235钢基体产生了塑性应变位错和局部的微裂纹,因此磨损进一步增加,磨损机制主要为疲劳磨损和磨粒磨损。
PEB/C/N试样的共渗层有效阻挡了Cl−对基体的腐蚀,磨损机制主要为磨粒磨损。
结论PEB/C/N试样在NaCl腐蚀介质中的耐腐蚀和耐磨性能得到明显提升。
关键词:低碳钢;等离子体电解;硼碳氮共渗;开路电位;耐腐蚀性;耐磨性中图分类号:TG156.8 文献标识码:A 文章编号:1001-3660(2023)06-0080-08DOI:10.16490/ki.issn.1001-3660.2023.06.008Tribo-Electrochemical Characteristics of PEB/C/NLayer on Low-Carbon SteelWANG Bin1, ZHOU Qian2,3, GAO Chuan-li2,3, LI Hui2,3, JIN Xiao-yue3, XUE Wen-bin2,3(1. Department of Basic Science, Shanxi Agricultural University, Shanxi Taigu 030801, China;2. Key Laboratory of Beam Technology and Materials Modification of Ministry of Education,College of Nuclear Science and Technology, Beijing Normal University, Beijing 100875, China;3. Institute of Radiation Technology, Beijing Academy of Science and Technology, Beijing 100875, China)ABSTRACT: Plasma electrolytic boriding (PEB) technology is an effective way to form iron borides on the steel surface.收稿日期:2022−05−04;修订日期:2022−10−26Received:2022-05-04;Revised:2022-10-26基金项目:山西省高等学校科技创新项目(2019L0389);国家自然科学基金(51671032,51071031)Fund:Technology and Innovation Fund of Shanxi Institution of Higher Education (2019L0389); National Natural Science Foundation of China (51671032, 51071031)作者简介:王彬(1987—),男,博士,副教授,主要研究方向为表面工程。
钠离子电池过渡金属硒化物负极材料的研究进展

㊀第56卷第3期郑州大学学报(理学版)Vol.56No.3㊀2024年5月J.Zhengzhou Univ.(Nat.Sci.Ed.)May 2024收稿日期:2023-08-30基金项目:国家自然科学基金青年项目(52202119)㊂第一作者:杨明醒(1995 ),男,硕士研究生,主要从事钠离子电池负极材料研究,E-mail:yy734778380@㊂通信作者:朱建华(1992 ),男,讲师,主要从事高功率储能材料研究,E-mail:jianhuazhu@㊂钠离子电池过渡金属硒化物负极材料的研究进展杨明醒,㊀朱建华(郑州大学㊀河南先进技术研究院㊀河南郑州450003)摘要:钠离子电池(SIBs)因其原材料来源丰富,在大规模储能领域具有较强的竞争力,有望成为锂离子电池的重要补充㊂负极材料是制约钠离子电池发展的关键问题㊂在众多的钠离子电池负极材料中,过渡金属硒化物(TMSs)有着高理论容量㊁低成本和环境友好的优点,被认为是有希望的候选材料㊂首先,阐明了TMSs 的钠储存机制㊂然后,阐述了TMSs 目前存在的首次库仑效率低㊁体积膨胀大㊁导电性差和多硒化物穿梭效应等问题㊂随后,讨论了相应的改进策略,并详细介绍了TMSs 在纳米结构设计㊁碳包覆㊁构建异质结和其他方面的最新研究进展㊂最后,进行了对TMSs 的总结和展望㊂关键词:钠离子电池;过渡金属硒化物;纳米结构设计;碳包覆;异质结中图分类号:TB333文献标志码:A文章编号:1671-6841(2024)03-0016-06DOI :10.13705/j.issn.1671-6841.2023202Research Progress on Transition Metal Selenide Anode Materialsfor Sodium-ion BatteriesYANG Mingxing,ZHU Jianhua(Henan Institute of Advanced Technology ,Zhengzhou University ,Zhengzhou 450003,China )Abstract :Due to the abundance of raw materials,sodium-ion batteries (SIBs)with strong competitive-ness in the field of large-scale energy storage were expected to be an important complement to lithium-ion batteries.The anode was a key issue restricting the development of sodium-ion batteries.Among various anode materials for SIBs,transition metal selenides (TMSs)were considered promising candidates be-cause of their high theoretical capacity,low cost,and environmental friendliness.Firstly,the sodiumstorage mechanism of TMSs was elucidated.The challenges of TMSs were discussed subsequently,such as low initial Coulombic efficiency,significant volume expansion,poor conductivity,and the polyselenide shuttle effect.Afterwards corresponding improvement strategies were explored,followed by a comprehen-sive overview of the latest research progress on TMSs,including nanostructure design,carbon coating,heterostructure construction,and other aspects.Finally,a summary and outlook for TMSs were provided.Key words :sodium-ion battery;transition metal selenide;nanostructure design;carbon coating;hetero-geneous structure0㊀引言随着化石燃料开采规模的不断扩大,地壳中煤炭㊁石油和天然气等化石能源的储量逐渐减少,这不仅导致化石燃料的枯竭,还给地球环境带来严峻的挑战[1]㊂近年来,为减少能源消耗和改善环境问题,清洁㊁环保㊁可持续的能源,如风能㊁太阳能㊁水能和潮汐能,引起了学术界的广泛关注㊂然而,这些能源往往具有不可预测性㊁容量不稳定性和间歇性等㊀第3期杨明醒,等:钠离子电池过渡金属硒化物负极材料的研究进展缺点,在实际应用中受到很大的限制[2]㊂为了更有效地利用这些清洁能源,必须开发大规模电化学储能(EES)系统㊂锂离子电池(LIBs)凭借其体积小㊁功能强大和寿命长的优点,在便携式电子设备和电动汽车(EV)领域得到了广泛应用,成为最成功的商业电化学技术之一[3]㊂然而,锂资源有限且成本高昂,而电动汽车销量的快速增长进一步推高了锂的成本[4]㊂因此,有必要探索适用的储能器件在未来的储能领域与锂离子电池共同担负起可持续绿色能源开发的重任㊂钠是地球上最丰富的金属元素之一,分布均匀,是仅次于锂的第二轻和第二小的碱金属,具有与锂相似的物理和化学特性㊂基于钠丰度以及与锂离子电池相似的工作原理,可充电钠离子电池(SIBs)是锂离子电池的理想互补器件[5]㊂当前,商业化的SIBs主要受到负极材料的限制㊂这是因为Na+的半径较大,无法直接应用于锂离子电池(LIBs)的石墨负极材料[6-7]㊂然而,SIBs 的正极材料,如类普鲁士蓝㊁层状过渡金属氧化物和聚阴离子化合物,在电化学性能方面接近商业化的锂铁磷酸盐(LiFePO4),几乎已经满足了商业应用的要求[8-10]㊂因此,开发适用于SIBs的负极材料对于SIBs的发展和商业化至关重要㊂在众多具有潜力的负极材料中,过渡金属硒化物(TMSs)由于其高容量㊁低成本和环境友好等优点而具有一定的优势㊂相比于同族的氧化物和硫化物,金属硒化物具有较高的电子导电性㊁较小的体积变化和良好的可逆性能[11]㊂此外,由于金属硒化物中的M Se键能较M O键和M S键更弱,金属硒化物的极化较小,电化学反应动力学更优异,倍率性能更好[12-13]㊂综上,过渡金属硒化物作为SIBs负极材料具有巨大的潜力和广阔的前景,并受到了广泛的关注㊂接下来,本文将重点介绍钠离子电池过渡金属硒化物负极材料的最新研究进展㊂1㊀过渡金属硒化物负极材料的储钠机制及现存挑战1.1㊀储钠机制相对于锡(Sn)㊁锑(Sb)和铋(Bi),过渡金属具有较低的活性,往往无法与Na进行合金化反应㊂因此,过渡金属硒化物的储钠机制通常为 插层-转化 机制㊂在放电过程中,Na+不断嵌入硒化物材料并发生转化反应,最终形成Na2Se和过渡金属颗粒㊂而在充电过程中,则发生相反的过程㊂上述机制目前已被多次证实㊂以CoSe2为例, Zhang等[14]通过XRD㊁SAED和近边缘X射线吸收光谱(XANES),系统地研究了CoSe2的钠储存机制㊂XRD揭示了Na+和CoSe2之间的转化反应过程㊂放电至0.5V后产生Co和Na2Se,二次充电至3.0V后出现单独的CoSe2,SAED的结果与XRD一致㊂随后,通过非原位XANES揭示了CoSe2的嵌入反应过程㊂基于上述结果,他们提出了CoSe2的可逆钠储存机制:CoSe2⇆Na x CoSe2⇆CoSe+Na2Se⇆Co+Na2Se㊂1.2㊀现存挑战尽管过渡金属硒化物具有许多优点,但距离商业化应用仍很遥远,其中存在的主要问题如下㊂(1)由于不稳定的固体电解质界面(SEI)层的形成,金属硒化物通常显示出较低的初始库仑效率(ICE)㊂(2)金属硒化物在充㊁放电过程中体积剧烈变化,导致电极的自粉碎和容量的快速衰退㊂(3)金属硒化物固有的低离子和电子电导率导致较差的倍率性能㊂(4)放电过程中形成的多硒化物中间体的 穿梭效应 会降低电化学性能㊂2㊀过渡金属硒化物负极材料现行优化策略2.1㊀纳米结构设计纳米级材料拥有比表面积大㊁反应活性高㊁应变能力强等优点㊂纳米材料能够有效缩短Na+的传输距离,从而获得优异的电化学性能[15-16]㊂通过对纳米材料的结构和形貌进行设计,可以进一步提升其电化学性能㊂该方法目前已被广泛应用㊂Yue等[17]基于硬-软-酸碱理论,分别使用Se粉和NaBH4作为Se源和还原剂,在室温下制备出直接在铜网上生长的Cu2Se纳米片㊂纳米片之间的间距有利于电解质的渗透并提供更多的活性位点,从而提高钠存储容量㊂为了稳定结构,他们随后在Cu2Se纳米片上引入导电聚合物聚吡咯(PPy)作为保形涂层,构建具有核壳结构的Cu2Se@PPy,进一步缓解了钠存储过程中活性材料的剧烈体积膨胀和剥落㊂Zhu等[18]通过多元醇溶液化学法成功合成了单晶CuSe2纳米立方材料(CuSe2NCs)㊂单晶CuSe2中原子的整齐排列可以促进钠离子的传输并减少离子扩散的能垒㊂纳米级立方体形貌有利于电解液的71郑州大学学报(理学版)第56卷渗透,缓解循环过程中的体积膨胀㊂密度泛函理论(DFT)计算表明CuSe 2NCs 具有低扩散能垒㊂其较少的副反应㊁增强的动力学特性和稳定的微观结构使CuSe 2NCs 电极各项电化学性能均优于无定形结构的CuSe 2纳米块,尤其初始库仑效率更是几乎达到了100%㊂Ren 等[19]以乙二醇为溶剂,通过溶剂热法制备了装饰还原氧化石墨烯的自组装3D FeSe 2纳米花(FeSe 2/rGO-EG)㊂乙二醇溶剂不仅可以作为还原剂,还可以用作结构导向剂来控制材料的成核和结晶,促进(110)晶面的生长,DFT 计算阐明了Na +在FeSe 2(110)晶面上具有较低的吸附能和迁移能垒,有利于Na +的嵌入和脱嵌㊂众多3D 花状纳米粒子均匀分布在石墨烯表面,形成特殊的3D 结构,可以缩短Na +传输路径并增加电极的比表面积,从而加速反应动力学㊂表1是对上述TMSs 纳米结构设计策略电化学性能的总结㊂表1㊀TMSs 纳米结构设计策略总结Table 1㊀Summary of nanostructure design modification strategy材料结构形貌制备方法比容量/(mAh ㊃g -1)循环圈数Cu 2Se 片状㊀铜网浸泡法263.52000CuSe 2立方体多元醇溶液化学法3441700FeSe 2花状㊀溶剂热法4001000㊀㊀注:1)Cu 2Se 比容量测试和循环圈数测试的电流密度均为10A /g;2)CuSe 2比容量测试和循环圈数测试的电流密度分别为50A /g 和10A /g;3)FeSe 2比容量测试和循环圈数测试的电流密度均为1A /g㊂2.2㊀碳包覆对于TMSs 充放电过程中存在的体积膨胀问题,利用碳材料对其进行包覆已被证实为一种有效且通用的方法㊂碳壳骨架不仅为TMSs 的膨胀提供了充足的缓冲空间,增强了电子电导率,并且锚定了部分多硒化物,从而抑制了穿梭效应㊂同时,这些碳基质避免了金属硒化物和电解质之间的直接接触,减少了副反应并提高库仑效率㊂热解金属有机框架(MOFs)前驱体是对金属颗粒实现碳包覆的一个通用方法[20]㊂以MOFs 为前驱体衍生的电极材料有着超大的比表面积,并且MOFs 衍生电极的多孔结构有效增加了Na +的活性位点,增强了其储钠能力[21-23]㊂同时,电极材料基体中碳的存在提高了电导率并增强了倍率性能㊂Liang 等[24]通过多层沸石咪唑酯骨架结构(ZIF)前驱体的逐步外延生长以及后碳化和硒化的工艺,成功设计并合成了多层蛋黄壳CoSe 2纳米十二面体,为钠离子的插入提供大量的活性位点㊂尽管MOFs 材料有着诸多优点,然而其在后续的化学合成过程中容易发生断裂和塌陷[25]㊂为了解决这一问题,选择性涂层已被证明是保护MOFs 材料的有效方法[26]㊂共价有机骨架(COF)以共价键为特征,表现出优异的化学和热稳定性,可以有效保护MOFs 在反应过程中免受结构损坏[27]㊂Chen 等[28]首次通过双框架设计制备了类似梭子的Fe 3Se 4/NC 结构㊂Fe 3Se 4/NC 源自涂着COF 涂层的MOF,具有大比表面积和高孔隙率,非常适合Na +的嵌入和脱嵌㊂COF 层有效解决了MOF 在剧烈合成和循环反应过程中结构损伤的问题㊂此外,NC 涂层的高电导率增加了Fe 3Se 4/NC 的电子和离子迁移速率,从而有利于提高倍率性能㊂Liu 等[29]以氯化钠为模板㊁葡萄糖为碳源㊁尿素为氮源制备了三维氮掺杂碳网络,首次创建了应力分散结构,并将CoSe 2与导电网络有效连接,成功地控制了其在导电网络上的分布程度㊂在CoSe 2@NC中,CoSe 2均匀锚定在NC 导电网络上,形成具有强界面相互作用的纳米结构,从而改善了电子传输并减少了体积变化,使其能够形成稳定的固态电解质界面膜(SEI)并减少死钠的量㊂传统碳包覆策略都依赖共轭非极性碳平面的位置,对极性TMSs 的固定和捕捉作用有限,因此提高碳对TMSs 颗粒的亲和力也同样至关重要㊂Yuan 等[30]在封闭的高压釜中对壳聚糖㊁尿素㊁Cu (NO 3)2和SeO 2组成的混合物进行水热处理,随后Cu 前体和Se /C 的混合物在惰性气氛中热解,形成Cu 2-x Se@3D-CN㊂值得指出的是,SeO 2不仅是形成连接三维大孔骨架的模板,而且是合成TMS 和形成Se C 铆接键的来源㊂该方法可将各种TMS 植入到氮掺杂的多孔碳上,并在3D 纳米结构混合体中形成强大的铆接Se C 键,使得碳基质与TMS 纳米颗粒具有很强的亲和力,即使在长期循环后也可以防止TMSs 纳米颗粒聚集和分离,从而确保结构完整性㊂碳基体的结构也被改变,成了更强的吸收电子单元来储存Na +,促进了Na +的扩散和转化㊂该方法为碳纳米结构植入金属硒化物提供了一个通用模板路线㊂表2对上述碳包覆策略进行了总结㊂81㊀第3期杨明醒,等:钠离子电池过渡金属硒化物负极材料的研究进展表2㊀TMSs碳包覆策略总结Table2㊀Summary of carbon coating modification strategy材料主体碳包覆法方法优势比容量/(mAh㊃g-1)循环圈数CoSe2热解MOFs材料结构灵活易于操控352.92000 Fe3Se4COFs材料涂覆MOFs COF层增强了MOF材料的结构稳定性333.51000CoSe2三维氮掺杂碳网络浸渍和硒化首次创建了应力分散结构,并成功控制硒化物在导电网络的分布程度318.04500Cu2-x Se混合物水热增强了碳对硒化物的亲和力,并提供了一个通用的模板路线311.0200㊀㊀注:1)CoSe2比容量测试和循环圈数测试的电流密度均为1A/g;2)Fe3Se4比容量测试和循环圈数测试的电流密度分别为12A/g和8A/g;3)CoSe2比容量测试和循环圈数测试的电流密度分别为1A/g和5A/g;4)Cu2-x Se比容量测试和循环圈数测试的电流密度均为1A/g㊂2.3㊀构建异质结近年来,半导体异质结材料在电化学储能领域得到了广泛的应用[31-32]㊂通过在单金属硒化物中引入新的金属元素,形成异质界面,提供了丰富的晶格畸变和缺陷[33]㊂异质界面通过强大的界面协同效应,可以促进金属硒化物局部的电子和离子转移,从而增强了反应动力学㊂特定的异质结构可以获得更多储钠活性位点㊂不同金属的氧化还原电位差异还导致了电化学反应的异步性,从而抑制了Na+嵌入和脱嵌过程中体积变化[33]㊂Cao等[34]通过水热法和原位硒化反应合成了球中球结构的(M Mn)Se/C(M=Co,Ni)㊂Co(Ni) Se2和MnSe晶体之间的异质界面增强了离子扩散动力学,提高了电导率,并且提供了大量的储钠反应位点㊂值得一提的是,这种特殊的异质结构构建的内置电场建立了高效的3D电子传输网络,从而实现了优异的电化学性能㊂在过渡金属中引入活性金属构建异质结,不仅能发挥双金属协同作用,同时有利于提升材料整体的电化学性能㊂Liu等[35]通过水热法和涂覆热解成功制备了MoSe2-Sb2Se3@C复合材料㊂因双金属硒化物的协同效应,所获得的MoSe2-Sb2Se3@C复合材料表现出良好的倍率性能和高循环稳定性㊂通过DFT计算验证了其基本机制,窄带隙和引入的内置电场导致异质结处费米能级和逸出功的差异加速了电子迁移㊂Liu等[36]构建了具有相同阳离子和阴离子的Fe3Se4/FeSe异质结构㊂DFT计算表明,FeSe和Fe3Se4之间的界面有利于提高离子电子电导率和结构稳定性㊂对比单独的Fe3Se4电极,Fe3Se4/ FeSe电极具有优异的循环稳定性和出色的倍率性能㊂表3列出了上述各个异质结的电化学性能㊂表3㊀TMSs构建异质结设计策略总结Table3㊀Summary of heterostructure construction strategy异质结构制备方法比容量/(mAh㊃g-1)循环圈数Co(Ni)Se2/MnSe水热法㊁原位硒化441.21000 MoSe2/Sb2Se3水热法㊁涂覆热解376.0500 Fe3Se4/FeSe静电纺丝㊁气相硒化417.41000㊀㊀注:1)Co(Ni)Se2/MnSe比容量测试和循环圈数测试的电流密度均为5A/g;2)MoSe2/Sb2Se3比容量测试和循环圈数测试的电流密度均为2A/g;3)Fe3Se4/FeSe比容量测试和循环圈数测试的电流密度分别为0.5A/g和5A/g㊂2.4㊀其他策略除了上述三种常见的改进策略外,还存在一些其他已被证实的可行方法,但目前对这些方法的研究较少,因此下面只简要介绍㊂2.4.1㊀电解质调节㊀电解质作为电池系统的重要组成部分,对电池的性能起着至关重要的作用㊂Chen等[37]研究了溶剂对FeSe2微球电化学性能的影响㊂当使用二甘醇二甲醚(DGM)作为唯一溶剂时,FeSe2微球具有优异的循环稳定性㊂在此之后,醚基电解质被广泛应用于TMS,表现出比碳酸酯电解质优越的电化学性能㊂2.4.2㊀电压窗口调节㊀通过电位窗口调节可以避免TMSs发生深度转化反应,从而减少体积变化,延长循环寿命㊂Ou等[38]研究了截止电压对CoSe2纳米棒储钠能力的影响㊂结果显示,在0.4~3.0V的窗口范围内,CoSe2发生了高度可逆的钠储存过程㊂相反,当截止电压调整为0.01V时,CoSe2的储钠过程变得不可逆,800次循环后,容量保持率从之前的92.8%降低至3.8%㊂可以看出,调整截止电压是提高TMSs电化学性能的有效策略㊂2.4.3㊀过渡金属掺杂㊀Sun等[39]通过对CoSe2进行Mn掺杂,扩大了其晶格结构和间隙体积,从而缓解这种材料中钠储存的固有缓慢动力学㊂在水热处91郑州大学学报(理学版)第56卷理过程中,Mn的掺杂量可以通过调节添加Mn的比例来调节㊂DFT计算表明,在Mn/Co=1ʒ4的原子比下,Mn的掺入可以使间隙体积膨胀136.9%㊂与纯CoSe2@CNS/CNF相比,Co0.8Mn0.2Se2@CNS/CNF 显示出了更优异的电化学性能㊂3 总结与展望作为大规模能量存储领域锂离子电池的实际补充,钠离子电池可以满足先进能量存储技术的需求,并为可持续发展做出贡献㊂因此,开发高性能的SIB电极材料具有重要意义㊂过渡金属硒化物由于其较大的理论容量被认为是SIB负极材料具有希望的候选者㊂在本文中,阐明了过渡金属硒化物负极材料的钠储存机制,并总结了该材料的当前问题,如低初始库仑效率㊁严重体积膨胀㊁导电性差和穿梭效应㊂随后对改进策略进行分类和讨论,包括纳米结构设计㊁碳包覆㊁异质结构构建和其他方法㊂尽管近年来对过渡金属硒化物的研究日益增多,但仍存在许多未解决的问题,表明该材料仍具有持续的研究价值㊂这里提出了三个展望:(1)醚基电解质的有限氧化稳定性限制了正极材料的选择,需要开发新型醚基电解质;(2)SEI膜的形成机制尚不清楚,需要进一步探索使用先进的原位表征技术和理论计算以提高初始库伦效率;(3)增加对全电池的研究以推动商业化㊂参考文献:[1]㊀LI Y M,LU Y X,ZHAO C L,et al.Recent advances ofelectrode materials for low-cost sodium-ion batteries to-wards practical application for grid energy storage[J].Energy storage materials,2017,7:130-151. [2]㊀UD DIN M A,LI C,ZHANG L H,et al.Recent pro-gress and challenges on the bismuth-based anode for sodi-um-ion batteries and potassium-ion batteries[J].Materi-als today physics,2021,21:100486.[3]㊀LIANG Y R,LAI W H,MIAO Z C,et al.Nanocompos-ite materials for the sodium-ion battery:a review[J].Small,2018,14(5):1702514.[4]㊀RAHIL A,PARTENIE E,BOWKETT M,et al.Investi-gating the possibility of using second-life batteries for gridapplications[J]].Battery energy,2022,1(3):20210001.[5]㊀XIE X C,SHUAI H L,WU X,et al.Engineering ultra-enlarged interlayer carbon-containing vanadium disulfidecomposite for high-performance sodium and potassium ionstorage[J].Journal of alloys and compounds,2020,847:156288.[6]㊀JACHE B,ADELHELM e of graphite as a highlyreversible electrode with superior cycle life for sodium-ionbatteries by making use of co-intercalation phenomena[J].Angewandte Chemie,2014,53(38):10169-10173.[7]㊀WEN Y,HE K,ZHU Y J,et al.Expanded graphite assuperior anode for sodium-ion batteries[J].Nature com-munications,2014,5:4033.[8]㊀LIU Q N,HU Z,CHEN M Z,et al.The cathode choicefor commercialization of sodium-ion batteries:layeredtransition metal oxides versus Prussian blue analogs[J].Advanced functional materials,2020,30(14):1909530.[9]㊀LIU Q N,HU Z,LI W J,et al.Sodium transition metaloxides:the preferred cathode choice for future sodium-ionbatteries?[J].Energy&environmental science,2021,14(1):158-179.[10]JIN T,LI H X,ZHU K J,et al.Polyanion-type cathodematerials for sodium-ion batteries[J].Chemical societyreviews,2020,49(8):2342-2377.[11]HAO Z Q,SHI X Y,YANG Z,et al.Developing high-performance metal selenides for sodium-ion batteries[J].Advanced functional materials,2022,32(51):2208093.[12]HAO Z Q,DIMOV N,CHANG J K,et al.Tin phos-phide-carbon composite as a high-performance anode ac-tive material for sodium-ion batteries with high energydensity[J].Journal of energy chemistry,2022,64:463-474.[13]ALI Z,ZHANG T,ASIF M,et al.Transition metal chal-cogenide anodes for sodium storage[J].Materials today,2020,35:131-167.[14]ZHANG K,PARK M,ZHOU L M,et al.Urchin-likeCoSe2as a high-performance anode material for sodium-ion batteries[J].Advanced functional materials,2016,26(37):6728-6735.[15]FANG Y J,YU X Y,LOU X W.Nanostructured elec-trode materials for advanced sodium-ion batteries[J].Matter,2019,1(1):90-114.[16]LIU Q N,HU Z,ZOU C,et al.Structural engineering ofelectrode materials to boost high-performance sodium-ionbatteries[J].Cell reports physical science,2021,2(9):100551.[17]YUE L C,WANG D,WU Z G,et al.Polyrrole-encapsu-lated Cu2Se nanosheets in situ grown on Cu mesh for highstability sodium-ion battery anode[J].Chemical engi-neering journal,2022,433:134477.[18]ZHU Q,XU A D,CHEN H M,et al.CuSe2nanocubes02㊀第3期杨明醒,等:钠离子电池过渡金属硒化物负极材料的研究进展enabling efficient sodium storage[J].ACS applied mate-rials&interfaces,2023,15(10):12976-12985. [19]REN M M,ZANG H T,CAO S L,et al.Effect of sol-vent on the crystal phase,morphology,and sodium stor-age performance of FeSe2[J].Journal of materials chem-istry A,2023,11(19):10435-10444. [20]XU X J,LIU J,LIU J W,et al.A general metal-organicframework(MOF)-derived selenidation strategy for insitu carbon-encapsulated metal selenides as high-rate an-odes for Na-ion batteries[J].Advanced functional mate-rials,2018,28(16):1707573.[21]YANG S H,PARK S K,KIM J K,et al.A MOF-media-ted strategy for constructing human backbone-like CoMoS3@N-doped carbon nanostructures with multiple voids as asuperior anode for sodium-ion batteries[J].Journal ofmaterials chemistry A,2019,7(22):13751-13761.[22]YUAN J J,LIU W,ZHANG X K,et al.MOF derivedZnSe-FeSe2/RGO nanocomposites with enhanced sodium/potassium storage[J].Journal of power sources,2020,455:227937.[23]LI J B,LI J L,YAN D,et al.Design of pomegranate-like clusters with NiS2nanoparticles anchored on nitrogen-doped porous carbon for improved sodium ion storage per-formance[J].Journal of materials chemistry A,2018,6(15):6595-6605.[24]LIANG H J,LI X T,LIU X L,et al.Epitaxial growthinduced multilayer yolk-shell structured CoSe2with pro-moting transport kinetics of sodium ion half/full batteries[J].Journal of power sources,2022,517:230729.[25]SUN W W,TANG X X,WANG Y.Multi-metal-organicframeworks and their derived materials for Li/Na-ion bat-teries[J].Electrochemical energy reviews,2020,3(1):127-154.[26]AWUAL M R,HASAN M M,ASIRI A M,et al.Clean-ing the arsenic(V)contaminated water for safe-guardingthe public health using novel composite material[J].Composites part B:engineering,2019,171:294-301.[27]ZHANG Q F,WEI H P,WANG L L,et al.AccessibleCOF-based functional materials for potassium-ion batteriesand aluminum batteries[J].ACS applied materials&interfaces,2019,11(47):44352-44359. [28]CHEN H Y,LIU Q M,CAO S Y.Carbon-coated ironselenide derived from double-framework as an advance an-ode for Na-ion battery[J].Journal of colloid and inter-face science,2023,652:619-626.[29]LIU H H,LI D,LIU H L,et al.CoSe2nanoparticlesanchored on porous carbon network structure for efficientNa-ion storage[J].Journal of colloid and interfacescience,2023,634:864-873.[30]YUAN J,YU B,PAN D,et al.Universal source-tem-plate route to metal selenides implanting on3D carbonnanoarchitecture:Cu2-x Se@3D-CN with Se-C bonding foradvanced Na storage[J].Advanced functional materials,2023,33(46):2305503.[31]YUE L C,ZHAO H T,WU Z G,et al.Recent advancesin electrospun one-dimensional carbon nanofiber struc-tures/heterostructures as anode materials for sodium ionbatteries[J].Journal of materials chemistry A,2020,8(23):11493-11510.[32]FENG J,LUO S H,YAN S X,et al.Rational design ofyolk-shell Zn-Co-Se@N-doped dual carbon architecturesas long-life and high-rate anodes for half/full Na-ion bat-teries[J].Small,2021,17(46):e2101887. [33]FANG Y J,LUAN D Y,LOU X W D.Recent advanceson mixed metal sulfides for advanced sodium-ion batteries[J].Advanced materials,2020,32(42):e2002976.[34]CAO S Y,LIU Q M,CHEN H Y,et al.A bimetallic in-duced enhanced3D electron transport network supportedby micro constrain area of balls-in-ball structure used forhigh performance sodium storage[J].Chemical engineer-ing journal,2023,470:144277.[35]LIU Y,YI Y H,NIU Z L,et al.Heterojunction-promo-ted sodium ion storage of bimetallic selenides encapsula-ted in a carbon sheath with boosted ion diffusion and sta-ble structure[J].ACS applied materials&interfaces,2022,14(5):6926-6936.[36]LIU J W,XIAO S H,LI X Y,et al.Interface engineer-ing of Fe3Se4/FeSe heterostructure encapsulated in elec-trospun carbon nanofibers for fast and robust sodium stor-age[J].Chemical engineering journal,2021,417:129279.[37]CHEN Y L,ZHANG J T,LIU H J,et al.Controlledsynthesis of FeSe2nanoflakes toward advanced sodiumstorage behavior integrated with ether-based electrolyte[J].Nano,2018,13(12):1850141.[38]OU X,LIANG X H,ZHENG F H,et al.In situ X-raydiffraction investigation of CoSe2anode for Na-ion stor-age:effect of cut-off voltage on cycling stability[J].Electrochimica acta,2017,258:1387-1396. [39]SUN J G,JI D X,YE H L,et al.Doping induced hier-archical lattice expansion of cobalt diselenide/carbonnanosheet hybrid for fast and stable sodium storage[J].Cell reports physical science,2020,1(7):100082.12。
唐浩林,

唐浩林,男,1981年2月生。
美国电化学协会学学生会员和中国太阳能协会会员。
2001年6月毕业于武汉理工大学应用化学专业;2001年进入材料复合新技术国家重点实验室攻读硕士和博士学位,主要从事燃料电池关键材料的研制工作,导师潘牧教授。
攻读硕士论文期间,在导师潘牧教授的指导下提出了采用静电自组装的方法制备燃料电池膜电极和直接甲醇燃料电池抗甲醇渗透质子交换膜的技术,在该方面进行了大量的基础性研究和技术开发工作,得到了教育部博士点基金和国家自然科学基金的资助,获得了良好的材料性能和国际评价。
美国材料研究协会(MRS)对发表专门评述介绍了该项工作。
自2004年攻读博士学位以来,主要负责质子交换膜燃料电池最核心材料——质子交换膜的开发工作,制备的质子交换膜具有自主知识产权,各项指标均处优于国际同类产品,目前正在进行该材料的中试工程化和连续性生产开发。
该项目的部分基础研究工作将得到国家自然科学基金重点项目的资助。
进入课题组5年来,先后参与了国家自然科学基金重点项目和面上项目、863项目、教育部博士点基金项目和湖北省攻关项目的研究,在燃料电池领域发表学术论文34篇,其中SCI收录期刊论文10余篇;申请和获得国家发明专利12项。
Publications:1 Mu Pan, Haolin Tang, San Ping Jiang, Zengcai Liu, Self-assembledmembrane-electrode-assembly of polymer electrolyte fuel cells, Electrochemistry Communication, 2005, 7(2):119~1242 Mu Pan, Haolin Tang, San Ping Jiang, Zengcai Liu, Fabrication and performanceof polymer electrolyte fuel cells by self-assembly of Pt nanoparticles, Journal of the Electrochemical Society, 2005, 152(6) A1081-A10883 Pan Mu, Tang Haolin, Mu Shichun and Yuan Runzhang. Synthesis ofPlatinum/Multi-Wall Carbon Nanotube Catalysts,Journal of Materials Research, 2004, 19(8): 2279~22844 Tang Haolin, Pan Mu, Mu Shichun and Yuan Runzhang, Electrostaticself-assembly Pd particles on NafionTM membrane surface to reduce methanol crossover, Chin. Sci. Bull. 2005, 50(4) 377-3795 Haolin Tang, Mu Pan, Sanping Jiang, Zhaohui Wan and Runzhang Yuan,Self-assembling multi-layer-Pd nanoparticles onto NafionTM membrane to reduce methanol crossover, Colloids and Surfaces A 262 (2005) 65–706 Haolin Tang, Mu Pan, San Ping Jiang, Zengcai Liu, Synthesis of Platinumnanoparticles and the self-assembled on Nafion membrane as catalyst coated membrane, Journal of chemical research 2005(7)449-4517 Haolin Tang, Mu Pan, San Ping Jiang and Yuan Runzhang, Modification ofNafionTM membrane to reduce methanol crossover via self-assembly Pd nano-particles, Mater. Lett. 59 (2005) 3766-37708 Tang Haolin, Pan Mu, Mu Shichun and Yuan Runzhang. Synthesis of PlatinumNanoparticles modified with Nafion and the Application in PEM Fuel Cell,Journal of wuhan university of technology, mater. Sci. Ed. 2004, 19(3): 7~99 Mu Shichun, Tang Haolin*, Wan Zhaohui., Pan Mu and Yuan Runzhang. Aunanoparticles self-assembled onto Nafion membranes for use as methanol-blocking barriers. Electrochem. Commun.,7 (2005) 1143–1147.10 San Ping Jiang, Lin Li, Zengcai Liu, Mu Pan, and Hao Lin Tang, Self-Assemblyof PDDA-Pt Nanoparticle/Nafion Membranes for Direct Methanol Fuel Cells, Electrochemical and Solid-State Letters, 2005, 8 (11) A574-A57611 Shi-chun Mu , Hao-lin Tang,Sheng-hao Qian, Mu Pan, Run-zhang Yuan,Hydrogen storage in carbon nanotubes modified by microwave plasma etching and Pd decoration, Carbon 44 (2006) 762–76712 Luo Zhiping, Li Daoxi, Tang Haolin*, Pan Mu and Ruan Runzhang, Degradationbehaviors of membrane-electrode-assembly materials in 10-cell PEMFC stack, International Journal of Hydrogen Energy, in Press, Available online 4 May 200613 Mu Shichun, Wang Xiaoen Tang Haolin*, Li Peigang, Lei Ming, Pan Mu, YuanRunZhang. A Self-Humidifying Composite Membrane with Self-Assembled Pt Nanoparticles for Polymer Electrolyte Membrane Fuel Cells, Journal of The Electrochemical Society, 2006, 153 (10) A1868-A187214 San Ping Jiang, Zengcai Liu, Hao Lin Tang and Mu Pan, Synthesis andcharacterization of PDDA-stabilized Pt nanoparticles for direct methanol fuel cells , Electrochimica Acta,51 (2006) 5721–573015 Haolin Tang*, Mu Pan, Mu Shichun, Zhaohui Wan and Runzhang Yuan,Performance of Direct Formic Acid Fuel Cell with Self-assembled PEMs, Journal of Fuel Cell Science and Technology, in press, 2006.16 Haolin Tang, Shenlong Wang, Mu Pan, San Ping Jiang and Yunzhang Ruan,Performance of direct methanol fuel cells prepared by hot-pressed MEA and catalyst-coated membrane (CCM), Electrochimica Acta, in press, now available at web.17 MU Shichun , Tang Haolin ,QIAN Shenghao, PAN Mu , YUAN Runzhang ,Performance of hydrogen storage of carbon nanotubes decorated with palladium, Trans. Nonferrous Met. Soc. China, 2004, 14(5)996~99918 唐浩林,潘牧,木士春,袁润章,阳离子修饰纳米Pt的合成及其在Nafion膜上的自组装行为研究,化学学报,2004,62(2)127~13019 唐浩林,潘牧,木士春,袁润章,修饰离子聚合度对膜-颗粒体系静电自组装的影响,无机化学学报,2004,20(2)128~13220 唐浩林,潘牧,许程,木士春,袁润章,Pt-Nafion/CNTs的合成与表征,电池,2005,35(1)43~4421 唐浩林,潘牧,木士春,袁润章,离子修饰纳米Pt的合成及其在FC中的应用,电池工业,2004,9(2)87~9022 唐浩林,潘牧,张东方,袁润章,Nafion聚离子修饰纳米Pt的合成及其影响因素,化学研究与应用,2004,16(3)314~31623 唐浩林,潘牧,木士春,袁润章,阳离子聚合物修饰的纳米Pt颗粒的合成与影响因素,应用化学,2004, 21(8)779~78224 唐浩林,潘牧,赵修建,溶胶凝胶法制备α-Al2O3纳米材料团聚控制研究新进展,材料导报,2002,16(9)44~45;25 唐浩林,潘牧,赵修建,铝酸盐光致发光材料的相组成与粒径,化学通报,2003(3)174~177;26 唐浩林,潘牧,赵修建,溶胶等离子喷射合成法制备α-Al2O3纳米材料,陶瓷学报,2002,23(1)22~25;27 唐浩林,潘牧,木士春,袁润章,静电自组装质子交换膜燃料电池用膜电极的光谱学分析,精细化工,2003,20(9)524~52728 唐浩林,徐琴,木士春,潘牧,袁润章,谢春华, PEM燃料电池用气体扩散层材料研究进展,电池工业, 2004, 9(5) 253~25729 李笑晖,罗志平,唐浩林, 杨洁,潘牧, 磺化SEBS 质子交换膜制备和性能的研究, 功能材料, 2005 , 36(8)1213~121630 宛朝辉,唐浩林,木士春,潘牧,袁润章, 改性全氟磺酸阻醇膜研究进展, 电池工业, 2005, 10(8) 245~24831 罗志平,唐浩林,木士春,潘牧,谢春华, 国产碳纤维纸合成PEMFC气体扩散层, 电池工业, 2005, 10(6) 137~13932 汪圣龙, 潘牧,唐浩林,气体扩散层性能参数测量方法研究进展, 电池工业, 2005, 10(1)38~4233 汪圣龙,杨绍军,潘牧,唐浩林,木士春, PTFE 载量对气体扩散层性能的影响,电池, 2004, 34(6)401~40234 沈春辉,唐浩林,潘牧,袁润章, 燃料电池用非氟复合质子交换膜的研究进展, 精细化工, 2004, 21(s) 64~6735 许程, 唐浩林,木士春, 潘牧, 袁润章, PEMFC用Pt/CNTs电催化剂研究进展,电源技术, 2004, 28(10) 652~65536 汪圣龙, 唐浩林,潘牧, 木士春,袁润章, 膜电极结构对质子交换膜电池性能的影响, 2003, 17(10) 37~40Patents1潘牧, 唐浩林,宛朝辉, 袁润章, 谢春华.一种质子交换膜燃料电池核心组件的制作方法. 中国发明专利,CN 200410060944.4 (已授权)2潘牧, 唐浩林,袁润章, 宛朝辉, 谢春华. 一种降低氟化磺酸型质子交换膜甲醇渗透率的方法. 中国发明专利,申请号:200410060944.4(已授权)3潘牧, 唐浩林,李道喜, 余军, 袁润章. 具有自增湿功能的多层纳米复合质子交换膜的制备方法,中国发明专利,CN 200410061104.X (已授权)4唐浩林,潘牧, 王晓恩, 何秀冲, 木士春, 袁润章. 一种多孔高分子增强质子交换膜的制备方法,中国发明专利,申请号:200510018578.05唐浩林,潘牧, 何秀冲, 王晓恩, 木士春, 袁润章. 袁润章采用碱金属离子交换的全氟磺酸树脂制备燃料电池用复合质子交换膜的方法,中国发明专利,申请号:200510018912.26木士春, 陈磊, 唐浩林,潘牧, 袁润章, 高温质子交换膜燃料电池用复合质子交换膜及制备方法, 中国发明专利,申请号:200510018749.X7唐浩林,潘牧,王红红,木士春,宛朝晖,袁润章,,一种亲疏水性可调的质子交换膜燃料电池用核心组件的制备方法,中国发明专利,申请号:2006100118633.08唐浩林,潘牧,王红红,木士春,宛朝晖,袁润章,,一种亲疏水性可调的质子交换膜燃料电池用膜电极的制备方法,中国发明专利,申请号:2006100118634.59唐浩林,刘珊珊, 王晓恩, 潘牧, 袁润章, 一种基于亲水性多孔聚四氟乙烯基体的复合质子交换膜的制备方法, 中国发明专利,申请号:200610019386.6 10唐浩林,何秀冲, 潘牧, 袁润章, 无机矿物——质子传导树脂插层复合质子交换膜及其制备方法, 中国发明专利,申请号:200610019182.211唐浩林,宛朝辉, 潘牧, 袁润章,一种燃料电池用核壳催化剂及其制备方法,中国发明专利,申请号:200610019298.612唐浩林,宛朝辉, 潘牧, 袁润章,一种高效的直接甲醇燃料电池阴极催化剂及其制备方法,中国发明专利,申请号:200610019303.3。
小学下册T卷英语第五单元测验试卷(有答案)

小学下册英语第五单元测验试卷(有答案)英语试题一、综合题(本题有100小题,每小题1分,共100分.每小题不选、错误,均不给分)1.Her _____ (音乐盒) plays a lovely tune.2.The first successful vaccine was developed for ________ (天花).3.My sister is a ______. She enjoys sewing.4.The concept of a multiverse suggests there may be multiple ______.5.The fish has tiny ______ (鳍) for swimming.6.Glass is an example of a(n) _______ material.7.We have a picnic in the ________.8. A _______ is a common houseplant.9.My friend is a ______. He loves to swim.10.The movie starts at ________.11.What do we call the act of exchanging goods and services?A. TradeB. CommerceC. BarterD. All of the above答案: D12.The tree has ______ leaves. (green)13. A ______ (温暖的天气) encourages plant growth.14.The ______ helps us learn about historical figures.15. A tortoise can live for over a ________________ (百年).16.The _____ (tree/house) is tall.17.How many seasons are there in a year?A. 2B. 3C. 4D. 5答案:C18.What is the term for the process of plants making their own food using sunlight?A. RespirationB. PhotosynthesisC. GerminationD. Pollination答案: B19.Saturn's rings are believed to be remnants of a destroyed ______.20. A ________ (热带植物园) showcases exotic species.21.My favorite place to go shopping is _______ (商场).22.My brother is a member of the ____ (robotics) club.23. A chemical reaction that absorbs heat is called ______.24.The ________ (文化遗产) is valuable to preserve.25.What do you call a person who writes plays?A. PlaywrightB. AuthorC. ScreenwriterD. Novelist答案:A26.My favorite game is ______ (Scrabble).27.We enjoy _____ (hiking) in the fall.28.The deer runs fast in the _______ (鹿在_______中跑得很快).29.I like to play ________ with my friends.30. A _______ (小蜥蜴) can change color to blend in.31.My friend is a ______. He enjoys sports.32.I have a toy ________ that can roll.33.My favorite actress is _______ (名字). 她的表演很 _______ (形容词).34.The _______ (Roman Empire) expanded across Europe, North Africa, and the Middle East.35.The ancient Romans established one of the first ________ (共和国).36.Daisy and lily are examples of ______ (花卉).37. A _______ is used to measure the amount of energy used in a circuit.38.Ceres is the largest object in the asteroid ______.39.What is the name of the famous American holiday celebrated on the fourth Thursday in November?A. ChristmasB. ThanksgivingC. New Year's DayD. Independence Day答案:B40.The wind is ___ (strong/light).41.What do we call the time when the sun rises?A. SunsetB. MidnightC. DawnD. Noon答案: C. Dawn42.My cat likes to sleep on the _________ (沙发).43.The cake is very ___. (delicious)44.The __________ is a famous structure in India. (泰姬陵)45.What do we call a young female deer?A. CalfB. FawnC. KidD. Cub答案: B46.The rabbit has powerful _______ (后腿) for jumping.47.The _______ (Peasants’ Revolt) occurred in England in 1381.48.The dog is ___ (friendly/scary).49.The scientist, ______ (科学家), studies how plants grow.50.The apples are ________ in the basket.51.The squirrel gathers _________ (食物) for winter.52.What do we call the study of weather?A. MeteorologyB. BiologyC. ChemistryD. Geography答案:A53.My sister enjoys writing ____.54.The girl loves to ________.55.________ (果醋) is made from fruit.56. A ______ (植物的) study can be fun.57.The reaction of an acid with a metal produces ______ gas.58.My grandma enjoys making __________ (传统美食) for the family.59.The _______ (The Civil Rights Movement) sought to eliminate racial inequality.60.The __________ (历史的传递方式) influence understanding.61.What is 100 divided by 10?A. 5B. 10C. 15D. 20答案:B62.根据图片,写出单词。
矿物加工工程专业英语词汇.

矿物加工工艺学(浮选部分)英文词汇floatation 浮选froth flotation 泡沫浮选direct flotation 正浮选reverse flotation 反浮选fineness of grinding 磨矿细度fractionation 分级mineral wettability 矿物润湿性mineral flotability 矿物的可浮性equilibrium contact angle 平衡接触角three phase interface 三相界面hydrophobicity of mineral 矿物的疏水性hydrophilicity of mineral 矿物的亲水性foam adhesion泡沫附着ionic lattice 离子晶格covalence lattice共价晶格surface inhomogeneity 表面的不均匀性oxidation and dissolution 氧化与溶解oxidizing agent 氧化剂reduction agent 还原剂surface modification of mineral 矿物的表面改性electric double layer 双电层ionization 电离adsorption 吸附electrokinetic potential电动电位point of zero charge 零电点isoelectric point 等电点collecting agent 捕收剂semi micelle adsorption 半胶束吸附exchange adsorption 交换吸附competitive adsorption 竞争吸附specific adsorption 特性吸附modifying agent 调整剂depressant 抑制剂activating agent 活化剂foaming agent 起泡剂hydrophilic group 亲水基团liberation degree 解离度polar group 极性基团nonpolar group 非极性基团sulphide ore 硫化矿物oxidized mineral 氧化矿物xanthate 黄药hydrolysis 水解medicamentous selectivity药剂的选择性catchment action捕收作用electrochemical action 电化学作用pyrite 黄铁矿calcite 方解石alkyl radical 烃基含氧酸organic amine 有机胺类carboxylate surfactant 羧酸盐kerosene 煤油amphoteric collector 两性两捕收剂alkyl radical sulfonate 烃基磺酸盐complex 络合物pH modifying agent pH调整剂long-chain molecule 长链分子chalcopyrite 黄铜矿galena 方铅矿blende 闪锌矿oxidized ore 氧化矿flocculant 絮凝剂non-hydronium flocculant 非离子型絮凝剂desorption 解吸air bladder 气泡solubility 溶解度specific surface area 比表面积mineral resources 矿源three phase air bladder 三相气泡ore magma electric potential 矿浆电位mixed potential model 混合电位模型freedom hydrocarbon diversification 自由烃变化electrostatic pull 静电引力intermolecular force 分子间力goethite 针铁矿semi micelle adsorption 半胶束吸附concentration of solution 溶液浓度flotation machine浮选机oxygenation 充气作用recovery 回收率concentrate grade 精矿品位handling capacity 处理能力air bladder collision气泡碰撞flotation column 浮选柱ore concentration dressing 富集作用floatation process 浮选工艺floatation speed 浮选速率flotation circuit 浮选流程granularity 粒度degree of fineness 细度pulp density 矿浆浓度water quality 水质backwater 回水middlings 中矿run of mine 原矿gangue 脉矿flotation principle flow浮选原则流程rate of divergence 分散程度dispersant 分散剂semiconductivity of mineral矿物半导性reagent removal agent 脱药剂矿物加工工艺学(重选部分)英文词汇gravity concentration 重力选矿Abkhazite 透闪石棉Amiantus 石棉acceptance operation 矿石预选Acclivity 斜面airborne dust 大气浮尘air conveying 风力输送amplitude of vibration 振幅ancillary mineral 伴生矿物apparent viscosity 视粘度artificial bedding 人工床层attle 废石average grain diameter 平均粒径axial motion 轴向运动backwash water 冲洗水backwater筛下水barite 菱镁蛇纹岩barren rock 脉石beach ore 砂矿bed separation 分层bevel angle 倾斜角buddle 淘洗盘buddle jig 动筛跳汰机buoyancy 浮力buoyant weight 悬浮重量Caplastometer 粘度计Centipoises 厘泊Centrifugal field 离心力场Centrifugal jig 离心跳汰机Circular 圆形跳汰机Centrifuge 离心机Classification efficiency 分级效率Classifier 分级机Classifier overflow 分级机溢流Classifier sand 分级机返砂Close sizing 窄级分级Claster of particles 颗粒群Coarse feed 粗粒给料Cyclone 水力旋流器Cassiterite 锡石Dilated 松散床层dimensionless parameter 无因次参数duplex table 双层摇床diaphragm jig 隔膜跳汰机dwindles out 尖灭film concentration 流膜选矿final velocity 末速度free settling particle 自由沉降颗粒free settling ratio 自由沉降比gravity concentrate 重选精矿gravity tailings 重选尾矿galena 方铅矿iron ore pellet 铁矿球团jig cycle 跳汰周期heavy liquid 重液heavy-media separator 重介质分选heavy-media suspension 重介质悬浮液hydraulic analysis 水力分析high-weir spiral classifier 高堰式螺旋分级机hindered settling 干涉沉降HMS-flotation method 重介质浮选联合分选Hydrocyclone 水力旋流器Laundering 溜槽选矿low- weir spiral classier 低堰式螺旋分级机medium recovery screen 介质回收筛meerschaum 海泡石menachanite 钛铁砂outer vortex 外螺旋线particle diameter 颗粒直径particle shape 颗粒形状particle size accumulation 粒度累积曲线partition size 分离粒度jigging 跳汰选矿regenerated dense medium 重介质再生sand table 矿砂摇床scalping screen 脱介筛setting vessel 沉降速度shaking table 摇床sieve compartment 筛网室simplex spiral 单螺旋分级机sinusoidal wave 单层摇床sizing analysis 粒度分析silica 硅石spherical particle 球形颗粒spheroid 似球形spindle 针状形spiral chute 螺旋溜槽spiral concentrator 螺旋选矿机stiction 静摩擦submerged spiral type classifler 沉没式分级机suction bailer 吸入作用table 摇床table riffle 摇床格条table circuit 摇床流程table tailing 摇床尾矿table flotation 台浮talcum 滑石taraspite 白云石wedge angle 锥角(100) weight 重力矿物加工工艺学(磁电选矿部分)英文词汇Mineral Processing Technology 矿物加工工艺学Principle of magnetism process 磁选原理Magnetic force 磁力Ratio magnetic force 比磁力Compete force 竞争力Mineral magnetism 矿物的磁性Atomic magnetism moment 原子磁矩Molecular magnetism moment 分子磁矩Magnetization & magnetic field 磁化和磁化磁场Magnetization intensity 磁化强度Ratio susceptibility 比磁化系数Diamagnetism 逆磁性Paramagnetism 顺磁性Ferromagnetism 铁磁性Magnetic domain 磁畴Revers ferromagnetism 反铁磁性Subferromagnetism 亚铁磁性Coercive force 矫顽力Remanence 剩磁Magnetization roasting 磁化焙烧Deoxidization roasting 还原焙烧Midlle roasting 中性焙烧Oxidation roasting 氧化焙烧Siderite 菱铁矿Hematite 赤铁矿Magnetite 磁铁矿Unhydrophite magnetization 疏水磁化Magnetic process equipment 磁选设备Feebleness magnetic separation machine 弱磁场磁选机Dry magnetic separation machine 干式磁选机Wet feebleness magnetic separation machine 湿式弱磁场磁选机High magnetic separation machine 强磁场磁选机High grads magnetic sparation machine 高梯度磁选机Supercondduct magnetic separation 超导电选Concentrator 选矿机Electrity process 电选Electrity concentrator 电选机Static separation 静电选矿Air-ionization separation 电晕分选Friction electric separation 摩擦电选Magnetic process practice 磁选实践Nonmetal ore 非金属矿Diamond process 金刚石选矿Heavy medium reclaim 重介质回收Primary concentrate 粗精矿Graphite gangue 石墨尾矿Kaolin magnetic process 高岭土磁选Block metal ore 黑色金属矿石Manganese ore magnetic process 锰矿石磁选Coloured metal & rare metal 有色金属和稀有金属Ilmenite 钛铁矿Rutile 金红石Zircon 锆英石Electric process practice 电选实践Tungstate 钨酸盐cassiterite 锡石hematite 赤铁矿gangue 脉石,废石,矸石magnet 磁铁,磁体,磁石conductor mineral 导体矿物silicate 硅酸盐diatomite 硅藻土hysteresis 磁滞现象magnetic core . 磁铁芯winding 绕组,线圈medium 介质electrophoresis 电泳screening 筛分magnetic field 磁场flux 磁通量ferromagnet 铁磁物质ferromagnetism 铁磁性reunite 团聚magnetic system 磁系magnetic agitate 磁搅动permanent magnet 永久磁铁solenoid magnet 螺管式磁铁pyrite 黄铁矿,硫铁矿limonite 褐铁矿reluctivity 磁阻率conduct 传导induce 诱导,感应,归纳astrict 束缚charge 电荷electric field .电场interfacial 界面的,面间的magnetism 吸引力electrode 电极,电焊条,电极Strontium & iron oxid 锶铁氧体Periodic magnetic field 交变磁场Pulsant magnetic field 脉动磁场Saturation 饱和stainless steel material 不锈钢材料polar distance 极距mica 云母quarte 石英stimulate magnetism 激磁magnetism circuit 磁路magnetic line of force 磁力线commutate quality 整流性Flatation reagent professional wordsAbsorption 吸收Absorption band 吸收光谱带Abstract 抽出,提取Abundance 丰富,丰度Accelerant 促进剂Acceptance 验收,接收Accumulate 积累,聚集Accuracy 准确度Acctate 醋酸盐Acctamide 乙酰胺Acid 酸,酸的Acid anion 酸性阴离子Acidation 酸化Acid depression 加酸抑制Acid hydrolysis 加酸水解Acintol 妥尔油制品Acrylic amide丙烯酰胺Activate 活化Activated adsorption活性吸附Activated molecule 活化分子Activated effect 活化作用Activator 活化剂,活性剂Acto 精制石油磺酸钠Acylamide 酰胺Addition 加添Adhere 粘附,附着Adhesion coefficient粘着系数Adhesive粘合剂Adhesive tension胶结张力界面吸引力Adion 吸附离子Adsorbate 吸附物Adsorbent 吸附剂Adsorption isotherm吸附等温线Adsorption layer吸附层Aero 美国氰胺公司的药剂品牌号Aerofloat 美国氰胺公司的黑药牌号Aerofloc 絮凝剂牌号Aerofroth 起泡剂牌号Aeromine 阳离子型表面活性剂Aero promoter促进剂牌号Aerosol 润湿剂牌号Aerosurf MG-98A 醚胺醋酸盐Agglomerant 团聚的凝结剂Agglomeration flotation团聚浮选Aggregate of large molecules大分子团Aiv-avid亲气的Aiv-mineral adhesion空气-矿物粘附Alamine胺的牌号Alcohol醇Alcohol frother 醇类起泡剂Aliphat- 妥尔油脂肪酸牌号Aliphatic alcohol 脂肪醇Aliphatic acid 脂肪酸Aliphatic amine 脂肪胺Aliphatic dydrocarbon脂肪烃Aliquat苯胺盐牌号Alkali 碱Alkaliuity 碱度,碱性Alkane 链烷,烷烃Alkoxy- 烷氧基Alkoxyamine 烷氧胺Alkoxy benzene烷氧基苯Alkyl- 烷基Alkyl alcohol sulfate 烷基醇硫酸盐Alkylamine 脂肪胺Alkylarsonic acid 烷基砷酸Alkylarylsulfonate 烷基芳基磺酸盐Aldyl hydroxamic acid 烷基羟污酸Alkyl phosphate 烷基磷酸盐Alkyl sodium sulfonate 烷基磺酸钠All-flotation approach 全浮处理法Allowance 允许,公差All-purpose 通用的Amine 胺的牌号Amino-acid 氨基酸Ammonia 氨Amphateric 两性的Amphoteric surfactant 两性表面活性剂Starch 淀粉Analysis 分析Angle角,角度Anion 阴离子Anion collector 捕收剂Anode 阳极,正极Anti-corrosive coating 防腐浮层Antifoamer 消泡剂Apparent hardness 表现硬度Applicability 活用性,适应性Aqua ion 水合离子Aquation 水合作用Armeen 胺的牌号Arosurf MG醚胺的牌号Affached bubble 粘附气泡Bagolax 甲基纤维素Barrett 煤焦杂酚油牌号Benzyl alcohol 苯甲醇Bromoform 溴仿,三溴甲烷Bubble 气泡,泡沫Bubbler 气泡器Butyl 丁基Butyl aerofoat丁基黑药Calcium oxide 氧化钙Capillary 毛细管,毛细作用Carbitol 卡必醇Carbohydrate 碳水化合物Cation 阳离子Cationic collector 阳离子捕收Cellulosice CMC 羧甲基纤维素Charge 电荷,充电Chelate 螯合物Chelate effect 螯合效应Chelation group 螯合基团Chemical adsorption 化学吸附Chemical ore processing 化学选矿Chloro acetic acid 氯乙酸Cohesion 粘结力凝聚力Collector 捕收剂Colloid 胶体Creosote oil杂酚油Critical PH value 临界PH值Concentrate grade精矿品位Concentration 精选、富集Cyanide 氰化物Daxad 烷基磺酸钠Deflocculator 反絮凝剂Defoamer 消泡剂Dehydrating agent 脱水剂Dehydrogenation 脱氧Delamine 妥尔油胺Dense liqued 重液Depressant 抑制剂Desorbent 解吸剂Deslimie 脱泥Desludging agent 脱泥剂Dicarboxylic acid 二羟酸Dodecylamine 十二胺,月桂胺Dodecylalcohol 十二烷醇Dodecyl amine-hydrochloride十二胺盐酸盐Dresinate 松脂酸皂捕收剂Dual cleaning 二重精选Duponol 烷基硫酸钠牌号Dust-allaying medium 防尘剂Dynamic balance 动态平衡Efficiency 效率,功效Electrochemical approach 电化学处理法Electro-kinetic potential 动电势Electrostatic attraction 静电吸引Emulsifying agent乳化剂Extract 提取,萃取Ferric sulfate 硫酸铁Flotation 浮选矿物加工工艺常用词汇(一)1选矿-Mineral separation (ore dressing) 2设计-Design3工艺-Process (craftwork)4初步设计-Initiative(preliminary) design 5流程-Flow(circuit)6流程图-flowsheet7施工设计-working design8设计方案-design project9粉碎-comminution10 磨矿-grinding11浮选-flotation12脱水-dehydration13干燥车间-drying shop14尾矿-tailing15精矿-concentrate16中矿-middles17精选-concentration18粗选-first concentration20选矿机-concentrator21矿浆ore pulp22分级-classification22磨矿-grinding23磨矿机-grinding mills24筛分-screen25粉碎-crush26筛分机-screener27粉碎机-crusher28颚式粉碎机-jaw crusher29圆锥粉碎机-cone crusher30冲击式粉碎机impact crusher31辊式粉碎机-crusher rolls32球磨机-ball mill33棒磨机-rod mill34自磨机-autogenous mills35震动筛-vibratory screener 36分级机-classification equipment 37浮选-flotation38浮选机-flotation equipment39重选- gravity concentration40特殊选-special selection41 浮选柱-flotation column42脱水机-spin-drier43干燥机-drier44总图-general chart45配置-deploy46运输-transport47环境保护-environment protect 48场址-field location(site)49布置-lay50设计资料-design information 51粉碎流程-comminution flow 52磨矿流程-grinding flow(circuit) 53浮选流程-flotation flow54金属矿-metallic mines55非金属矿-non-metallic mines 56闭路-close circuit(loop)57闭路流程-close flow58开路-cut circuit(loop)59开路流程-cut flow60废水-liquid waste61粉尘-powder62噪声-yawp63污染-contamination64沉淀-form sediment65净化-decontaminate66输送-transportation67矿石-ore68物料-material69给矿-feed ores70给料-feed stuff71设备-equipment72方案-project73标高-elevation74通道-passage75维修-maintain76检查-check77操作-operation78化验-test、assay79检测-examine80坡度-gradient81起重机-crane82堆积-accumulation83细粒-granule、fine84粗粒-coarse85尾矿坝-tailing dam86矿仓-feed bin(storehouse)87粉矿仓-crushing pocket88产品仓-product bin(storehouse) 89砂泵-pump90立式泵-stand pump91卧式泵-horizontal pump92耐酸泵-acid-proof pump93耐碱泵-alkali-resistant pump94勘察-reconnaissance95地形-landform96工程-engineering97设计步骤design process98规模-scale99选矿厂-concentrating mill100设计内容design content(二)1 comminution-粉碎2 comminution engineering-粉碎工程3粉碎机-comminuter4粉碎动力学-comminution kinetics 5筛分曲线图-screen analysis chart 6筛孔-screen aperture7筛面-screen area8筛条screen bar9筛框-screen box10筛选厂-screen building11筛分机生产能力screen capacity 12筛分槽-screen cell13筛布-screen cloth14筛分screen classification15筛孔-screen hole 16筛分车间-screenhouse17筛分分析-screen analysis18滚筒筛-screening-drum19筛分效率-screening efficiency20筛分速率-screening rate21筛网-screen mesh22筛制、筛比、筛序-screen scale23筛孔尺寸-screen size24套筛-screen set25筛序-screen size gradation26筛余物screen tailings27筛下产品-screen throughs(underflow.undersize) 28可碎性crushability29可碎性系数-crushability factor30碎矿仓-crushed ore pocket31粉碎产品-crushed product32粉碎粒度-crusher size33粉碎腔-crushing cavity34粉碎厂-crushing plant35粉碎系数-crushing coefficient36粉碎工段-crushiong section37助磨剂-grinding aid38磨球-grinding ball39 磨矿负荷-grinding charge40磨矿效率-grinding efficiency41磨矿-grinding ore42磨砾-grinding pebble43磨碎能力-grinding property44研磨试验grinding test45磨矿设备-grinding unit46磨矿速度-grinding rate47磨矿功率-grinding power48磨矿车间-grinding plant49可磨性-grindability50可磨性指数-grindability index51可磨性指标-grindability rating52可磨性试验-grindability test53研磨工-grinder54磨工车间-grindery55磨矿动力学-grinding kinetics56粉碎能-crushing energy57粉碎机给矿口-crushing mouth58粉碎面-crushing face59粉碎力-crushing force60粉碎机进料口-crusher throat61筛分动力学-screen kinetics62选厂矿仓-mill bin63 选厂中矿mill chats64选厂配置mill configuration65磨过的矿石-milled ore66磨机给料-mill feeder67选厂给矿-mill-head68研磨作用-milling action69磨机衬里mill liner70入选品位milling grade71入选品位矿石milling-grade ore 72磨矿机milling-grinder73细碎、精磨-milling grinding74磨矿介质-milling medium75磨矿法-milling method76选矿作业-milling operation77选矿厂-milling plant78选厂矿泥-milling slime79选厂厂址-mill site80磨机负荷-mill load81选矿工(工长)millan82磨机需用功率-mill power draft 83选矿质量控制mill puality control 84选矿取样-mill sampling85磨机外壳-mill shell86磨机矿浆-mill slurries87磨石-millstone88选矿厂储矿仓mill-storage89选厂尾矿-mill tail90选矿用水-mill water91磨矿机溶液-mill solution92选矿厂建筑师-millwright93分级沉淀-class setting94矿粉-mineral fine95分级-classification96分级溢流-classifier overflow97分级返砂-classifier sand98分级机-classifier99分级筛-classifying screen100分级箱-classifying box(三)1品位-grade2精矿品位-concentrate grade3尾矿品位-tailing grade4尾矿场-tail area(pile)5尾矿仓-tailing bin6尾矿滤饼-tailing cake 7尾矿坝-tailing dam8尾矿池-tailing pond(pit)9取样-taking cut(sampling)10滑石talc11蓝晶石-talc blue12 试样缩分-sample division13 分样器-sample divider14精矿取样-concentrate sampling15中矿取样-middles sampling16尾矿取样-tailing sampling17浓缩-thickening18精矿浓缩-concentrate thickening19选矿流程-concentrating circuit20精选机-concentrating mcching21试样缩分-sample reduction (splitting)22矿物组成-mineralcomposition23矿物组分-mineral constituent24矿床-mineral depost25矿物-mineral26选矿方法mineral dressing method27选矿厂-concentrating mill28选矿ore dressing,mineral separation29矿物分析-mineral analysis30矿物组合-mineral association31 试样袋-sample sack32矿床-deposit33矿物岩相facies34矿物纤维-mineral fiber35固、气界面-mineral-air interface36固、液界面-mineral-water interface37固、气、液接触mineral-air-water contact38矿物颗粒-grain39矿物鉴定-mineral identification40矿物资源-interest41矿物解离-mineralliberation42矿物特性mineral character43矿物储量-mineral reserve44矿物(成分)检验mineral logical examination 45扑收剂-Minerec,flotigan,46精矿回收率concentrate recovery47中矿回收率middles recovery48精选concentration49附着精矿气泡concentratr-loaded bubble50精选机-concentrating maching51分选判据-concentration criterion52富集比-concentration factor53选矿摇床-concentration table54选厂流程concentrator flow5选厂流程图concentrator flow sheet56试样品位-sample grade57絮凝剂-flocculant58絮凝-floculate59絮凝物-flocs60絮凝浮选floc flotation61絮凝作用flocculation62浮选机flotation unit63浮选剂- flotation agent64整排浮选机flotation bank65浮选槽- flotation cell66浮选能力flotation capacity67浮选精矿- flotation concentrate68浮选尾矿flotation rejects69浮选中矿- flotation middles70浮选设备flotation equipment71浮选泡沫-flotation froth72浮选动力学flotation kinetics73浮选浸出法- flotation leaching method74浮选厂flotation mill75浮选油-flotation oil76浮选矿浆- flotation pulp77浮选速度-flotation rate78浮选试验flotation test79单槽浮选机- flotation unit cell80浮选摇床- flotation table81摇床浮选- flotation tabling82起泡剂Flotol83流程图-flow line84工艺流程图-flow process chart (flow sheet) 85可选(洗)性-washability86可选性特性- washability characteristic87可选性曲线- washability curve88可选性指数- washability number89可选性试验- washability test90可浮性-flotability91可浮性曲线-flotability curve92粒度特性-granularity93粒度分级试验grading test94结构-texture95构造-tectonic(structural)96致密结构-compact texture97斑状结构porphyritic texture98 粒度分析-granularmetric analysis 99采样-sample collecting100分样器-sample divider1矿石Ore2可选性Separability3地质Geology4 选厂Concentrating mills5勘探Prospecting6脉石Gangue7选别方法Concentrating methods 8脉石矿物Gangue mineral9矿产资源Minerals resources10矿物Mineral11试样Sample12取样Assay Sampling13制样Preparation of samples14矿床Deposit15矿样Mineral samples16矿物学Mineralogy17物质组成Material composition18化学分析Chemical analysis19结构Texture20氧化矿Oxide ore21硫化矿Sulfide ore22金属矿物Metallic mineral23非金属矿物Nonmetallic mineral 24石墨Graphite25破碎Comminution26颚式粉碎机Jaw crushers27对辊机Roll crushers28筛分Screening29混合矿石Mixed ores30粒度Particle size31粒度分析Particle size analysis32选别产品Concentrating products33筛分试样Screening samples34爆破Blow35采矿Mining36粗粒Coarse-grain37细粒Fine-grain38矿浆Ore pulp39矿浆浓度Pulp density40 矿石特性Ore characteristics41结构特性Texture characteristics 42矿物分析Mineral analysis43物相分析Phase analysis44光谱分析Spectrum analysis45可磨性Grindability46硅酸盐Silicate47显微镜Microscope48磨碎Grinding49精矿Concentrate50中矿Middling51尾矿Tailing52磁选Magnetic separation53电选Electrical separation54重选Gravity concentration55重介质选Heavy medium separation56硅酸盐矿物Silicate minerals57矿物组成Mineral composition58品位Grad59矿物岩相Phases60 岩相分析Lithofacies analysis61矿物组合Mineral association62矿物鉴定Mineral identification63矿物解离Mineral liberation64构造Structure65斑状结构porphyritic texture66致密结构compact texture,dense structure 67 精矿品位Concentrate grade68尾矿品位Tailing grade69精选Cleaning70扫选scavenging;扫选机scavenger,71精矿回收率Concentrate recovery72中矿回收率Middling recovery 73浮选泡沫Flotation froth74絮凝Flocculate75絮凝剂Flocculant76絮凝浮选Flocculation flotation77浮选机flotation unit, flotation machine 78浮选药剂Flotation agent79絮凝物Flocs80絮凝作用Flocculation81选别流程Concentrating flow82浮选槽Flotation cell83浮选精矿Flotation concentrate84浮选尾矿Flotation tailing85浮选中矿Flotation middling86单槽浮选机Flotation unit cell87浮选试验Flotation test88起泡剂Frother89可浮性Flotability90浮选设备Flotation equipment91浮选速度Flotation rate92捕收剂collector93浮选能力Flotation capacity94闭路试验closed circuit test95条件试验Factor test96开路试验Open circuit test97活化剂Activator98棒磨机rod mill99球磨机ball mill100调整剂Regulating agent4) beach sand —n. sand ore from beach.〖海滨砂矿〗5) magnetite —n. a mineral with chemical formula Fe3O4〖磁铁矿〗6) quartz —n. a mineral chemical formula SiO2〖石英〗7) gangue —n. refuses from mining or ore dressing〖脉石矿物〗8) valuable minerals —n. some minerals which can be used in industries〖有用矿物〗9) wolframite —n. a mineral with chemical formula (Fe,Mn)WO4〖黑钨矿〗10) cassiterite —n. a mineral with chemical formula SnO2〖锡石〗11) diamagnetics —n. some substances which can repel the effect from magnetic field〖抗磁性物质,抗磁质〗〖=diamagnetic substance〗12) paramagnetic substance —n. some substances which can appeal the effect from magnetic field〖顺磁性物质,顺磁质〗13) rutile —n. a mineral with chemical formula TiO2〖金红石〗14) monasite —n. a mineral with chemical formula (Ce,La)PO4, consists of Rare Earth(RE)〖独居石〗15) siderite —n. a mineral with chemical formula FeCO3〖菱铁矿〗16) pyrrhotite —n. a mineral with chemical formula FeS〖磁黄铁矿〗17) chromite —n. a mineral with chemical formula FeCr2O4〖铬铁矿〗18) ferromagnetic substance —n. some substances which have susceptibility to magnetic forces and retain some magnetism when removed from the field〖铁磁性物质,铁磁质〗19) ilmenite —n. a mineral with chemical formula FeTiO3〖钛铁矿〗20) hematite —n. a mineral with chemical formula Fe2O3〖赤铁矿〗13) specular hematite ore —n. a mineral which has chemical composition Fe2O3〖镜铁矿〗14) scheelite —n. a mineral which has chemical composition CaWO4〖白钨矿〗15) asbestos —n. soft, fibrous, grey mineral substance that can be made into fire-proof fabrics or solid sheeting and used as a heat-insulating material〖石棉〗16) molybdenum-bearing minerals —n. a mineral containing molybdenum〖含钼矿物〗5) sulphur —n. light-yellow non-metallic element (symbol S) that burns with a bright flame and a strong smell, used in medicine and industry〖硫〗10) kaolin —n. fine white clay used in making porcelain, etc.〖高岭土、陶土〗Part 5 Magnetic Intensities Required to Extract Minerals〖第五部分分选矿物所需要的磁场强度〗Technical Terms〖技术术语〗1) alabandite —n. a mineral which has chemical composition MnS〖硫锰矿〗2) ankerite —n. a mineral which has chemical composition Ca(Mg,Fe,Mn)[CO3]2〖铁白云石〗3) apatite —n. a mineral which has chemical composition Ca5[PO4]3(F,Cl,OH)〖磷灰石〗4) bastnasite —n. a mineral which has chemical composition (Ce,La)[CO3]F〖氟碳铈矿〗5) biotite —n. a mineral which has chemical composition K(Mg,Fe)3[AlSi3O10](OH)2〖黑云母〗6) braunite —n. a mineral which has chemical composition 3Mn2O3·MnSiO3〖褐锰矿〗7) chromite —n. a mineral which has chemical composition FeCr2O4〖铬铁矿〗8) chrysocolla — n. a mineral which has chemical composition CuSiO3·2H2O〖硅孔雀石〗9) columbite —n. a mineral which has chemical composition (Fe,Mn)(Nb,Ta)2O6〖铌铁矿〗10) davidite —n. a mineral which has chemical composition (Fe,U,Ce)(Ti,Fe)3(O,OH)7〖铀钛磁铁矿〗11) epidote —n. a mineral which has chemical composition Ca2(Al,Fe)3[Si2O7][SiO4]O(OH)〖绿帘石〗12) euxenite —n. a mineral which has chemical composition (Y,Ca,Ce,U,Th)(Nb,Ta,Ti)2O6〖黑稀金矿〗13) ferberite —n. a mineral which has chemical composition FeWO4〖钨铁矿〗14) franklinite —n. a mineral which has chemical composition ZnFe2O4〖锌铁尖晶石〗15) garnet —n. a mineral which has chemical composition (Ca,Mg,Fe,Mn)3 (Al,Fe,Mn,Cr,Ti)2(SiO4)3〖石榴石〗17) hematite —n.iron oxide which has chemical formula Fe2O3〖赤铁矿〗18) hornblende —n. a mineral which has chemical composition (Ca,Mg,Al,Fe2,Mn,Na2,Na2,K2)SiO3〖角闪石〗19) ilmenite —n. a mineral which has chemical composition FeTiO3〖钛铁矿〗20) ilmeno-rutile —n. a mineral which has chemical composition TiO2·Fe(Nb,Ta)2O6〖黑金红石〗21) limonite —n. a mineral which has chemical composition Fe2O3·nH2O〖褐铁矿〗22) maghemite —n. a mineral which has chemical composition Fe2O3〖磁赤铁矿〗23) magnetite —n. a mineral which has chemical composition Fe3O4〖磁铁矿〗24) martite —n. a mineral which has chemical composition Fe2O3〖假象赤铁矿〗25) monazite —n. a mineral which has chemical composition (Ce,La,Y,Th)[PO4]〖独居石〗26) muscovite —n. a mineral which has chemical composition KAl2[AlSi3O10](OH)2〖白云母〗27) olivine —n. a mineral which has chemical composition (Fe,Mg)2[SiO4]〖橄榄石〗28) pyrochlore(or:pyrochlorite) —n. a mineral which has chemical composition (Ca,Ce)2Nb2(O,F)7〖黄绿石〗29) pyrolusite —n. a mineral which has chemical composition MnO2〖软锰矿〗30) pyrrhotite —n. a mineral which has chemical composition Fe1-x S〖磁黄铁矿〗31) renierite —n. a mineral which has chemical composition Cu3(Fe,Ge,Zn)(As,S)4〖硫铜锗矿〗32) rhodochrosite —n. a mineral which has chemical composition MnCO3〖菱锰矿〗33) rhodonite —n. a mineral which has chemical composition MnSiO3〖蔷薇辉石〗34) samarskite —n. a mineral which has chemical composition (Y,Er,Ce,U,Ca,Fe,Pb,Th)(Nb,Ta,Ti,Sn)2O6〖铌钇矿〗35) siderite—n. a mineral which has chemical composition FeCO3〖菱铁矿〗36) staurolite —n. a mineral which has chemical composition FeAl4[SiO4]2O2(OH)2〖十字石〗37) serpentine —n. a mineral which has chemical composition Mg6(Si4O10) (OH)8〖蛇纹石〗38) tantalite(or:tantaline) —n. a mineral which has chemical composition (Fe,Mn)Ta2O6〖钽铁矿〗39) titaniferous-magnetite —n. a mineral which has chemical composition (Fe,Ti)3O4〖含钛磁铁矿〗40) tourmaline —n. a mineral which has chemical composition (Na,Ca)(Mg,Al)6[B3Al3Si6(O,OH)30]〖电气石〗41) uraninite —n. a mineral which has chemical composition UO2〖沥青铀矿〗42)wolframite —n. a mineral which has chemical composition (Fe,Mn)WO4〖黑钨矿〗43) xenotime —n. a mineral which has chemical composition YPO4〖磷钇矿〗1) niobium —n. an element for Nb〖铌〗2) tantalum —n. an element for Ta〖钽〗3) liquid helium —n. helium in liquid state〖液氦〗2) sulphide —n. a kind of mineral containing sulphur〖硫化物、硫化矿〗3) oxidised minerals —n. 〖氧化矿〗Part 11 Collectors〖第十一部分捕收剂〗Technical Terms〖技术术语〗1) grease tabling —n. a special separation way 〖油膏摇床分选〗2) creosote —n. tar oil〖焦馏油〗3) skim —vt. throw away or discharge〖撇去、刮去〗4) hydrophilic —adj. a property that polar mineral surface react strongly with water molecules〖亲水的〗5) polarity —n.state in which there are two opposite, conflicting or contrasting qualities, principles or tendencies〖极性〗6) galena —n. 〖方铅矿PbS〗7) covellite —n. 〖铜蓝、蓝铜矿CuS〗8) bornite —n. 〖斑铜矿Cu5FeS4〗9) chalcocite —n.〖辉铜矿Cu2S〗11) stibnite —n.〖辉锑矿Sb2S3〗12) argentite —n.〖辉银矿Ag2S〗13) bismuthinite —n.〖辉铋矿Bi2S3〗14) millerite —n.〖针镍矿NiS〗15) cobaltite —n.〖辉砷钴矿CoAsS〗16) arsenopyrite —n.〖毒砂FeAsS〗17) pyrite —n.〖黄铁矿FeS2〗18) sphalerite —n.〖闪锌矿ZnS〗19) orpiment —n.〖雌黄As2S3〗20) pentlandite —n.〖镍黄铁矿(Fe,Ni)9S8〗21) realgar —n.〖雄黄As4S4〗22) barite —n.〖重晶石BaSO4〗23) anhydrite —n.〖硬石膏,无水石膏CaSO4〗24) gypsum —n.〖石膏CaSO4 ·2H2O〗25) anglesite —n.〖铅矾PbSO4〗26) cerrusite —n.〖白铅矿PbCO3〗27) malachite —n.〖孔雀石Cu2[CO3](OH)2〗28) azurite —n.〖蓝铜矿Cu3[CO3]2(OH)2 〗29) wulfenite —n.〖钼铅矿PbMoO4〗30) fluorite —n.〖萤石CaF2〗31) calcite —n.〖方解石CaCO3〗32) witherite —n.〖毒重石BaCO3〗33) magnesite —n.〖菱镁矿MgCO3〗34) dolomite —n.〖白云石CaMg(CO3)2〗35) apatite —n.〖磷灰石Ca5(PO4)3(F,Cl,OH)〗36) scheelite —n.〖白钨矿CaWO4〗37) smithsonite —n.〖菱锌矿ZnCO3〗38) rhodochrosite —n.〖菱锰矿MnCO3〗39) siderite —n.〖菱铁矿FeCO3〗40) monazite —n.〖独居石(Ce,La,Y,Th)PO4〗41) hematite —n.〖赤铁矿Fe2O3〗42) magnetite —n.〖磁铁矿Fe3O4〗43) goethite —n.〖针铁矿Fe2O3·H2O〗44) chromite —n.〖铬铁矿FeCr2O4〗45) ilmenite —n.〖钛铁矿FeTiO3〗46) corundum —n.〖刚玉,金刚砂Al2O3〗47) pyrolusite —n.〖软锰矿MnO2〗48) limonite —n.〖褐铁矿Fe2O3·nH2O〗49) borax —n.〖硼砂Na2[B4O5](OH)4·8H2O〗50) wolframite —n.〖黑钨矿(Fe,Mn)WO4〗51) columbite —n.〖铌铁矿(Fe,Mn)(Nb,Ta)2O6〗52) tantalite —n.〖钽铁矿(Fe,Mn)(Ta,Nb)2O6 〗53) rutile —n.〖金红石TiO2〗54) cassiterite —n.〖锡石SnO2 〗55) zircon —n.〖锆石ZrSiO4〗57) hemimorphite —n.〖异极矿Zn4[Si2O7](OH)2·H2O〗58) beryl —n.〖绿柱石Be3Al2[Si6 O18]〗59) feldspar —n.〖长石〗60) sillimanite —n.〖硅线石Al2[SiO4]O〗61) garnet —n.〖石榴石〗62) quartz —n.〖石英SiO2〗Part 12 Anionic Collectors〖第十二部分阴离子捕收剂〗Technical Terms〖技术术语〗1) oxyhydryl collectors —n. 〖烃基含氧酸类捕收剂〗2) oleic acid —n. 〖油酸〗3) sodium oleate —n. 〖油酸钠〗4) linoleic acid —n. 〖亚油酸〗5) kyanite —n. 〖蓝晶石〗6) ethyl —n. 〖乙基〗7) isopropyl —n.〖异丙基〗8) isobutyl —n.〖异丁基〗9) amyl —n.〖戊基〗10) hexyl —n.〖己基〗11) xanthate —n.〖黄药,黄原酸盐〗12) sulphydryl collectors —n.〖硫代化合物捕收剂〗Part 13 Cationic Collectors 〖第十三部分阳离子捕收剂〗Technical Terms〖技术术语〗1) amine collectors —n. 〖胺类捕收剂〗2) electrical double layer —n. 〖双电层〗3) collecting power —n. collective strength〖捕收力〗4) barite —n. 〖重晶石BaSO4〗5) carnallite —n. 〖光卤石〗6) sylvite —n. 〖钾盐〗7) alkaline —adj. 〖碱性的〗8) alkali earth metal—n. 〖碱土金属〗Part 14 Frothers 〖第十四部分起泡剂〗Technical Terms〖技术术语〗1) bubble attachment —n. 〖气泡附着〗2) stability —n. 〖稳定性〗3) liquid phase —n. 〖液相〗4) sulphide —n. 〖硫化物〗5) collecting launder —n. 〖收集槽〗6) mineral processing plant —n. 〖选矿厂〗7) heteropolar —adj. 〖异极性的〗8) hydroxyl —n. 〖羟基–-OH〗9) carboxyl —n. 〖羧基–-COOH〗10) carbonyl —n. 〖羰基=C=O〗11) amino group —n. 〖氨基–-NH2〗12) sulpho group —n. 〖磺酸基–-OSO2OH〗13) pine oil —n. 〖松节油〗。
柠檬酸改性球形活性炭对氨气吸附性能的影响

化工进展Chemical Industry and Engineering Progress2024 年第 43 卷第 2 期柠檬酸改性球形活性炭对氨气吸附性能的影响郭迎春,梁晓怿(华东理工大学化工学院,上海 200237)摘要:以沥青基球形活性炭为载体,采用等体积浸渍法将不同浓度的柠檬酸负载到活性炭孔隙内。
通过固定床动态吸附装置评价柠檬酸改性活性炭对氨气吸附性能的影响。
采用扫描电子显微镜、X 射线衍射仪、傅里叶变换红外光谱和氮气吸脱附对改性前后活性炭的理化特性进行表征分析。
结果表明,当柠檬酸负载量为60%(质量分数)时,改性活性炭对氨气的吸附性能最佳,氨气防护时间为194min ,单位活性炭的氨气吸附容量为42.8mg/mL (66.8mg/g ),是未改性活性炭吸附容量的24倍;吸附剂的总比表面积和孔体积以及pH 都随着柠檬酸负载量的增加不断减小,其中微孔的比表面积和孔体积与柠檬酸负载量的相关系数R 2分别为0.9944和0.9842;柠檬酸的利用率随着负载量的增加不断减少,氨气与柠檬酸反应生成柠檬酸铵,产物主要沉积在微孔内,微孔对氨气吸附至关重要。
关键词:活性炭;柠檬酸;复合材料;固定床;吸附;氨气中图分类号:X511 文献标志码:A 文章编号:1000-6613(2024)02-1082-07Effect of citric acid modification on the spherical activated carbon ’sammonia adsorption performanceGUO Yingchun ,LIANG Xiaoyi(School of Chemical Engineering, East China University of Science and Technology, Shanghai 200237, China)Abstract: The pitch-based spherical activated carbon was used as support to load different amounts of citric acid into its pores by equivalent-volume impregnation method. The effect of citric acid modification on the activated carbon ’s ammonia adsorption was evaluated by a fixed bed dynamic adsorption device. The physicochemical properties of activated carbon before and after modification were characterized by SEM, XRD, FTIR, nitrogen adsorption and desorption. The results showed that the modified activated carbon had the best ammonia adsorption performance when the load of citric acid was 60%. The ammonia protection time was 194min, and the ammonia adsorption capacity was 42.8mg/mL (66.8mg/g), which was 24 times that of unmodified activated carbon. The total specific surface area, pore volume and pH of the adsorbent decreased with the increase of citric acid loading, and the correlation coefficients R 2 between the specific surface area and pore volume of the adsorbent, and citric acid loading were 0.9944 and 0.9842, respectively. As the loading capacity increased, the utilization rate of citric acid decreased. Ammonia reacted with citric acid to produce ammonium citrate. The products were mainly deposited in micropores, which were crucial for ammonia adsorption.Keywords: activated carbon; citric acid; composites; fixed-bed; adsorption; ammonia研究开发DOI :10.16085/j.issn.1000-6613.2023-0290收稿日期:2023-02-28;修改稿日期:2023-05-08。
阴极等离子体电解沉积铁镍
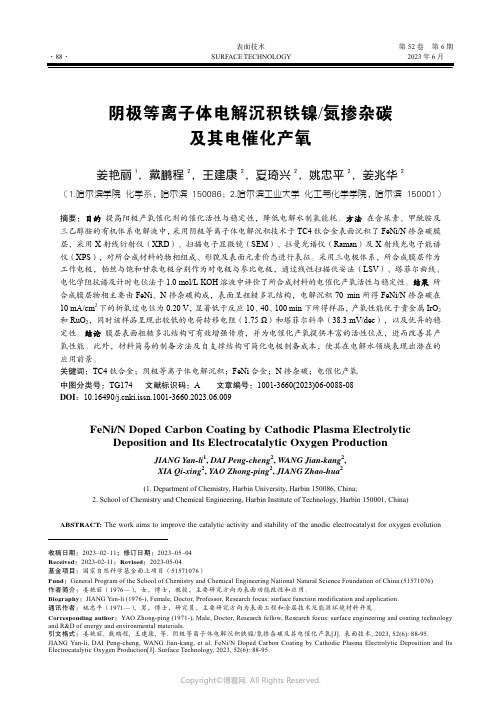
表面技术第52卷第6期阴极等离子体电解沉积铁镍/氮掺杂碳及其电催化产氧姜艳丽1,戴鹏程2,王建康2,夏琦兴2,姚忠平2,姜兆华2(1.哈尔滨学院 化学系,哈尔滨 150086;2.哈尔滨工业大学 化工与化学学院,哈尔滨 150001)摘要:目的提高阳极产氧催化剂的催化活性与稳定性,降低电解水制氢能耗。
方法在含尿素、甲酰胺及三乙醇胺的有机体系电解液中,采用阴极等离子体电解沉积技术于TC4钛合金表面沉积了FeNi/N掺杂碳膜层,采用X射线衍射仪(XRD)、扫描电子显微镜(SEM)、拉曼光谱仪(Raman)及X射线光电子能谱仪(XPS),对所合成材料的物相组成、形貌及表面元素价态进行表征。
采用三电极体系,所合成膜层作为工作电极,铂丝与饱和甘汞电极分别作为对电极与参比电极,通过线性扫描伏安法(LSV)、塔菲尔曲线、电化学阻抗谱及计时电位法于1.0 mol/L KOH溶液中评价了所合成材料的电催化产氧活性与稳定性。
结果所合成膜层物相主要由FeNi、N掺杂碳构成,表面呈粗糙多孔结构,电解沉积70 min所得FeNi/N掺杂碳在10 mA/cm2下的析氧过电位为0.20 V,显著低于反应10、40、100 min下所得样品,产氧性能优于贵金属IrO2和RuO2,同时该样品呈现出较低的电荷转移电阻(1.75 Ω)和塔菲尔斜率(38.3 mV/dec),以及优异的稳定性。
结论膜层表面粗糙多孔结构可有效增强传质,并为电催化产氧提供丰富的活性位点,进而改善其产氧性能。
此外,材料简易的制备方法及自支撑结构可简化电极制备成本,使其在电解水领域表现出潜在的应用前景。
关键词:TC4钛合金;阴极等离子体电解沉积;FeNi合金;N掺杂碳;电催化产氧中图分类号:TG174 文献标识码:A 文章编号:1001-3660(2023)06-0088-08DOI:10.16490/ki.issn.1001-3660.2023.06.009FeNi/N Doped Carbon Coating by Cathodic Plasma ElectrolyticDeposition and Its Electrocatalytic Oxygen ProductionJIANG Yan-li1, DAI Peng-cheng2, WANG Jian-kang2,XIA Qi-xing2, YAO Zhong-ping2, JIANG Zhao-hua2(1. Department of Chemistry, Harbin University, Harbin 150086, China;2. School of Chemistry and Chemical Engineering, Harbin Institute of Technology, Harbin 150001, China)ABSTRACT: The work aims to improve the catalytic activity and stability of the anodic electrocatalyst for oxygen evolution收稿日期:2023–02–11;修订日期:2023–05–04Received:2023-02-11;Revised:2023-05-04基金项目:国家自然科学基金面上项目(51571076)Fund:General Program of the School of Chemistry and Chemical Engineering National Natural Science Foundation of China (51571076)作者简介:姜艳丽(1976—),女,博士,教授,主要研究方向为表面功能改性和应用。
乙酸改性活性炭纤维对SO2吸附性能的研究

河南科技Henan Science and Technology 化工与材料工程总第812期第18期2023年9月乙酸改性活性炭纤维对SO2吸附性能的研究罗涛朋(南昌师范学院化学与食品科学学院,江西南昌330032)摘要:【目的】通过温和的改性手段,提升活性炭纤维(ACF)对SO2的吸附能力,制备一种低成本、绿色环保的吸附材料。
【方法】以ACF为前驱体,采用不同体积分数乙酸浸泡和不同时长200℃热处理进行改性,并测试了改性后的活性炭纤维材料对SO2的吸附能力,初步探究了改性机理。
【结果】ACF经200℃高温处理后,碳纤维出现裂纹,表面更加粗糙,使材料比表面积增加,提升了对SO2的吸附能力,其中热处理3h的结果最佳。
乙酸溶解浸泡改性后,会增加材料表面的酸性含氧官能团,提升材料对SO2的吸附性能,通过不同体积分数乙酸处理,探究得出体积分数4%的乙酸溶液处理过的ACF脱硫效率最高。
【结论】本研究方法相较于传统改性方法更加温和、环保,为活性炭纤维的改性研究提供参考。
关键词:活性炭纤维;浸泡改性;吸附中图分类号:TQ342.742文献标志码:A文章编号:1003-5168(2023)18-0075-04 DOI:10.19968/ki.hnkj.1003-5168.2023.18.016Study on Adsorption Properties of Acid Modified Activated CarbonFiber for SO2After Soaking in Acetic LUO Taopeng(College of Chemistry and Food Science,Nanchang Normal University,Nanchang330032,China)Abstract:[Purposes]The adsorption capacity of activated carbon fiber(ACF)for SO2was enhanced through mild modification techniques,resulting in the preparation of a cost-effective and environmen⁃tally friendly adsorbent material.[Methods]The precursor ACF was subjected to a200℃heat treatment and soaked in varying concentrations of acetic acid.The adsorption capacity of modified activated carbon fiber material for SO2was tested,and the reaction mechanism was preliminarily explored.[Findings]The results revealed that after treated at200℃,the carbon fibers exhibited cracks and a rougher surface, thereby increasing the specific surface area of the material and enhancing its adsorption capacity for SO2. Heat treatment for3hours yielded optimal outcomes.After acetic acid dissolution soaking modification, the acidic oxygen-containing functional groups on the surface of the material will be increased,which can improved the adsorption performance of the material for SO2.Experimental results indicated that ACF treated with a4%acetic acid solution exhibited the highest desulfurization efficiency.[Conclu⁃sions]The aforementioned method is more gentle and environmentally friendly compared to the tradi⁃tional modification approach,thereby offering a valuable reference for activated carbon fiber modifica⁃tion.Keywords:activated carbon fiber;soaking;adsorption收稿日期:2023-08-02基金项目:江西省教育厅科研项目(GJJ2202025、GJJ2202026);南昌师范学院校级科研项目(22XJZR01、22XJZR03)。
活性炭疏水

第53卷第5期表面技术2024年3月SURFACE TECHNOLOGY·1·研究综述活性炭疏水/超疏水改性技术研究进展庄怡凡,刘星宇,花颢轩,苏一凡,侯泽钟,周张恒,季延正,焦玄,余新泉*,张友法*(东南大学 a.材料科学与工程学院 b.江苏省先进金属材料高技术研究重点实验室,南京 211189)摘要:活性炭具有丰富的孔隙结构和表面官能团,广泛用于吸附污染环境中的各种有机物。
由于活性炭表面富含亲水官能团,在潮湿环境下将优先吸附水分子,从而大幅度降低了对目标有机物的吸附容量,因此如何改善活性炭的防潮防水性能是其应用过程中亟待解决的问题。
大量研究表明,活性炭经疏水/超疏水改性后,可有效隔绝水汽,显著提高其防潮、防水性能,这种特殊性能使疏水/超疏水活性炭在诸多领域都有着极高的应用前景和市场价值。
基于活性炭的吸附机理,从活性炭的物理结构和表面化学性质2个角度出发,概述了活性炭的来源和形貌,着重介绍了不同的疏水/超疏水改性技术,包括高温加热处理、负载金属氧化物、有机分子改性、有机硅涂层、有机无机复合超疏水涂层的改性制备方法及相应的优缺点,并在此基础上分析了不同改性技术对活性炭的防潮、防水能力及吸附目标有机物的影响。
进一步讨论了疏水/超疏水活性炭在不同领域(例如废水处理、废气处理及电化学催化等)的实际应用效果,并指出了现有技术存在的局限性,最后对活性炭的发展进行了展望。
关键词:活性炭;有机物吸附;超疏水;防潮;防水中图分类号:TQ31 文献标志码:A 文章编号:1001-3660(2024)05-0001-17DOI:10.16490/ki.issn.1001-3660.2024.05.001Progress in Hydrophobic or SuperhydrophobicModification of Activated CarbonZHUANG Yifan, LIU Xingyu, HUA Haoxuan, SU Yifan, HOU Zezhong, ZHOU Zhangheng,JI Yanzheng, JIAO Xuan, YU Xinquan*, ZHANG Youfa*(a. School of Material Science and Engineering, b. Jiangsu Key Laboratory for Advanced Metallic Materials,Southeast University, Nanjing 211189, China)ABSTRACT: Activated carbon is one of the most widely used absorbents in the field of removing various organic substances in polluted environments, due to its abundant pore structure and a high degree of surface reactivity. However, the surface chemical properties of activated carbon are rich in hydrophilic functional groups. This means that when water molecules and organic pollutants coexist, they compete for active sites on the surface of activated carbon. In this situation, activated carbon tends to preferentially adsorb water molecules, which drastically diminishes its adsorption capacity of the desired organic pollutants. This negatively affects its efficiency when used in humid environments. Therefore, how to收稿日期:2023-02-27;修订日期:2023-05-08Received:2023-02-27;Revised:2023-05-08基金项目:国家自然科学基金(52071076)Fund:National Natural Science Foundation of China (52071076)引文格式:庄怡凡, 刘星宇, 花颢轩, 等. 活性炭疏水/超疏水改性技术研究进展[J]. 表面技术, 2024, 53(5): 1-17.ZHUANG Yifan, LIU Xingyu, HUA Haoxuan, et al. Progress in Hydrophobic or Superhydrophobic Modification of Activated Carbon[J]. Surface Technology, 2024, 53(5): 1-17.*通信作者(Corresponding author)·2·表面技术 2024年3月improve the moisture and water resistance of activated carbon is an urgent problem that needs to be resolved in the application of an complex multi-component environment. A large number of studies have shown that modifying activated carbon to be hydrophobic or superhydrophobic can effectively tackle the thorny problem.Currently, numerous researchers both domestically and internationally have studied primary hydrophobic or superhydro-phobic methods of activated carbon, which include high-temperature heating treatment modification, loaded metal oxide modification, organic molecular modification, silicone coating modification, and organic-inorganic composite superhydrophobic coating modification. Those methods have been confirmed effectively isolate water vapor from being adsorbed into the pore space by activated carbon. Researchers have also found that improving the moisture and water resistances of the modified activated carbon is of great significance. Such developments demonstrate the practical application value and far-reaching prospects of hydrophobic or superhydrophobic activated carbon. This review aims to highlight the progress made in research on the application of hydrophobic or superhydrophobic modification of activated carbon. Based on the mechanism of activated carbon's adsorption and desorption for organic matter, the significant factors that affected its adsorption performance were carefully discussed. It was demonstrated that the physical structures and surface chemistry properties had a significant impact on activated carbon's absorption performance. These influencing characteristics included the specific surface area, pore size and distribution, functional groups and more. To better understand those properties, firstly, an overview of different types and sources of activated carbon was provided. The specific surface area, pore distribution, and surface functional groups of the carbon depended on its type and source, which ultimately affected its absorption performance. To further optimize the activated carbon's performance, there were different hydrophobic or superhydrophobic modification preparation techniques available.Those methods enhanced the hydrophobic and alter the surface chemistry properties leading to increased adsorption capacity.According to those modification preparation techniques, the change in the adsorption capacity of the modified activated carbon to the target organic matter in a humid environment before and after modification were further explored. In addition, the actual application effect of hydrophobic or superhydrophobic activated carbon in a wide variety fields, such techniques as wastewater removal organic treatment, exhaust removal organic gas treatment, and the newly developed electrochemical catalytic hydrophobic treatment were discussed. At the same time, their respective advantages and disadvantages, as well as the current limitations that need to be resolved for the existing technologies were pointed out. In the end, this article examined the challenges faced by activated carbon and explored the potential development trends for the hydrophobic or superhydrophobic modification of activated carbon, which showed great promise. It was hoped to provide theoretical reference and basis for the research and development of hydrophobic or superhydrophobic activated carbon in the field of realistic application.KEY WORDS: activated carbon; organic adsorption; superhydrophobic; moisture-proof; waterproof活性炭(Activated Carbon, AC)拥有丰富的孔隙结构,具有优异的吸附性能,且价格低廉,已广泛用于吸附各种环境中的污染有机物。
- 1、下载文档前请自行甄别文档内容的完整性,平台不提供额外的编辑、内容补充、找答案等附加服务。
- 2、"仅部分预览"的文档,不可在线预览部分如存在完整性等问题,可反馈申请退款(可完整预览的文档不适用该条件!)。
- 3、如文档侵犯您的权益,请联系客服反馈,我们会尽快为您处理(人工客服工作时间:9:00-18:30)。
Department of Chemical and Environmental Engineering National University of Singapore, 10 Kent Ridge Crescent, Singapore 129791, Singapore b Institute of High Performance Computing, 1 Science Park Road, [01 -01, The Capricorn, Singapore 117528, Singapore Received 19 February 2003; accepted 5 May 2003
* Corresponding author. Tel.: 1 65-6874-8092; fax: 1 656779-1936. E-mail address: checjp@.sg (J.P. Chen).
carbon had much higher adsorption of cadmium, lead and zinc. Lakov et al. [3] modified activated carbons with 3-methyl-1-phenyl-pyrazolone-5 (HMPP). The copper adsorption capacity was enhanced by 50% even though the specific surface area of the carbon decreased by 80%. More recently, Monser and Adhoum [4] reported that, when tetrabutyl ammonium iodide (TBA) or sodium diethyl dithiocarbamate (SDDC) were applied onto an activated carbon, a substantial improvement in Cu, Zn and Cr removal capacity was achieved. It has been reported that citric acid has a higher binding affinity to copper ions [5]. It is biodegradable and widely used in the food and pharmaceutical industries. As a result, it was selected for the modification of a commercially available granular activated carbon in this study. The main objective of this paper was to compare the adsorption performance of citric acid-modified activated carbons with the unmodified activated carbons. The copper adsorption equilibrium and kinetics were determined. Langmuir and Freundlich isotherm equations were employed to quantify the adsorption equilibrium. The effect of solution pH on
0008-6223 / 03 / $ – see front matter 2003 Elsevier Ltd. All rights reserved. doi:10.1016 / S0008-6223(03)00197-0
Байду номын сангаас980
J.P. Chen et al. / Carbon 41 (2003) 1979–1986
copper ion adsorption behaviour was examined. An intraparticle diffusion model was used to investigate the adsorption mechanisms.
2. Experimental
2.1. Materials
pure nitrogen gas overnight at a temperature of 150 o C to remove any contaminants as well as moisture that might be present on the activated carbon surfaces. The surface morphology of the activated carbon samples was visualized by scanning electron microscopy (JEOL, JSM-5600V, Japan). SEM enables the direct observation of any surface microstructure changes in the activated carbons that would have occurred due to the citric acid modification. Potentiometric titration experiments were carried out with an automatic titrator (Metrohm 716 DMS Titrino, Switzerland). A 0.25-g amount of activated carbon was added to a conical flask containing 50 ml of 0.1 M sodium nitrate as background ionic strength. The solution was shaken for 48 h on a shaker. The titration was carried out using 0.1 M NaOH and 0.1 M HNO 3 solutions.
Carbon 41 (2003) 1979–1986
Surface modification of a granular activated carbon by citric acid for enhancement of copper adsorption
J. Paul Chen a , *, Shunnian Wu b , Kai-Hau Chong a
Abstract In this study, citric acid was used to modify a commercially available activated carbon to improve copper ion adsorption from aqueous solutions. The carbon was modified with 1.0 M citric acid, followed by an optional step of reaction with 1.0 M sodium hydroxide. It was found that the surface modification reduced the specific surface area by 34% and point of zero charge (pH pzc ) of the carbon by 0.5 units. Equilibrium results showed that citric acid modification increased the adsorption capacity to 14.92 mg Cu / g, which was 140% higher than the unmodified carbon. Higher initial solution pH resulted in higher copper adsorption. The chemical surface modification adversely affected the copper adsorption rate. Adsorption kinetic mechanisms were investigated with an intraparticle diffusion model. It was found that the modification did not change both external diffusion and intraparticle diffusion. 2003 Elsevier Ltd. All rights reserved.
In this study, Fitrasorb 200 (Calgon, Pittsburgh, PA, USA) was used. Copper chloride dihydrate from J.T. Baker (Phillipsburg, USA), and sodium nitrate, lead nitrate, sodium hydroxide, sodium acetate, acetic acid, hydrochloric acid, nitric acid and citric acid monohydrate from Merck (Germany) were used. All chemicals were of reagent grade. Solution pH was adjusted by 0.1 M HCl or 0.1 M NaOH. The citric acid has a chemical formula of HOOC–CH 2 –COH(COOH)–CH 2 –COOH, indicating that it is a small organic compound rich in carboxylic groups. The concentration of metal ions was measured using inductively coupled plasma emission spectroscopy (ICPES) (Perkin-Elmer Optima 3000, USA). Samples were acidified with concentrated nitric acid, and filtered with a 0.45-mm Whatman Autovial filter (USA) before analysis. Solution pH was measured using an Accumet basic pH meter (Fisher Scientific, USA).