土木外文翻译原文和译文
土木工程 外文文献翻译
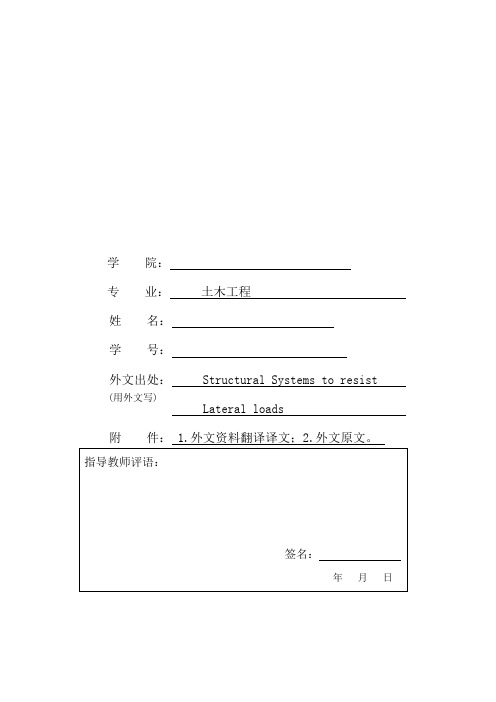
学院:专业:土木工程姓名:学号:外文出处: Structural Systems to resist (用外文写)Lateral loads附件: 1.外文资料翻译译文;2.外文原文。
附件1:外文资料翻译译文抗侧向荷载的结构体系常用的结构体系若已测出荷载量达数千万磅重,那么在高层建筑设计中就没有多少可以进行极其复杂的构思余地了。
确实,较好的高层建筑普遍具有构思简单、表现明晰的特点。
这并不是说没有进行宏观构思的余地。
实际上,正是因为有了这种宏观的构思,新奇的高层建筑体系才得以发展,可能更重要的是:几年以前才出现的一些新概念在今天的技术中已经变得平常了。
如果忽略一些与建筑材料密切相关的概念不谈,高层建筑里最为常用的结构体系便可分为如下几类:1.抗弯矩框架。
2.支撑框架,包括偏心支撑框架。
3.剪力墙,包括钢板剪力墙。
4.筒中框架。
5.筒中筒结构。
6.核心交互结构。
7. 框格体系或束筒体系。
特别是由于最近趋向于更复杂的建筑形式,同时也需要增加刚度以抵抗几力和地震力,大多数高层建筑都具有由框架、支撑构架、剪力墙和相关体系相结合而构成的体系。
而且,就较高的建筑物而言,大多数都是由交互式构件组成三维陈列。
将这些构件结合起来的方法正是高层建筑设计方法的本质。
其结合方式需要在考虑环境、功能和费用后再发展,以便提供促使建筑发展达到新高度的有效结构。
这并不是说富于想象力的结构设计就能够创造出伟大建筑。
正相反,有许多例优美的建筑仅得到结构工程师适当的支持就被创造出来了,然而,如果没有天赋甚厚的建筑师的创造力的指导,那么,得以发展的就只能是好的结构,并非是伟大的建筑。
无论如何,要想创造出高层建筑真正非凡的设计,两者都需要最好的。
虽然在文献中通常可以见到有关这七种体系的全面性讨论,但是在这里还值得进一步讨论。
设计方法的本质贯穿于整个讨论。
设计方法的本质贯穿于整个讨论中。
抗弯矩框架抗弯矩框架也许是低,中高度的建筑中常用的体系,它具有线性水平构件和垂直构件在接头处基本刚接之特点。
土木工程英语文献原文及中文翻译

Civil engineering introduction papers[英语原文]Abstract: the civil engineering is a huge discipline, but the main one is building, building whether in China or abroad, has a long history, long-term development process. The world is changing every day, but the building also along with the progress of science and development. Mechanics findings, material of update, ever more scientific technology into the building. But before a room with a tile to cover the top of the house, now for comfort, different ideas, different scientific, promoted the development of civil engineering, making it more perfect.[key words] : civil engineering; Architecture; Mechanics, Materials.Civil engineering is build various projects collectively. It was meant to be and "military project" corresponding. In English the history of Civil Engineering, mechanical Engineering, electrical Engineering, chemical Engineering belong to to Engineering, because they all have MinYongXing. Later, as the project development of science and technology, mechanical, electrical, chemical has gradually formed independent scientific, to Engineering became Civil Engineering of specialized nouns. So far, in English, to Engineering include water conservancy project, port Engineering, While in our country, water conservancy projects and port projects also become very close and civil engineering relatively independent branch. Civil engineering construction of object, both refers to that built on the ground, underground water engineering facilities, also refers to applied materials equipment and conduct of the investigation, design and construction, maintenance, repair and other professional technology.Civil engineering is a kind of with people's food, clothing, shelter and transportation has close relation of the project. Among them with "live" relationship is directly. Because, to solve the "live" problem must build various types of buildings. To solve the "line, food and clothes" problem both direct side, but also a indirect side. "Line", must build railways, roads, Bridges, "Feed", must be well drilling water, water conservancy, farm irrigation, drainage water supply for the city, that is direct relation. Indirectly relationship is no matter what you do, manufacturing cars, ships, or spinning and weaving, clothing, or even production steel, launch satellites, conducting scientific research activities are inseparable from build various buildings, structures and build all kinds of project facilities.Civil engineering with the progress of human society and development, yet has evolved into large-scale comprehensive discipline, it has out many branch, such as: architectural engineering, the railway engineering, road engineering, bridge engineering, special engineering structure, waterand wastewater engineering, port engineering, hydraulic engineering, environment engineering disciplines. [1]Civil engineering as an important basic disciplines, and has its important attributes of: integrated, sociality, practicality, unity. Civil engineering for the development of national economy and the improvement of people's life provides an important material and technical basis, for many industrial invigoration played a role in promoting, engineering construction is the formation of a fixed asset basic production process, therefore, construction and real estate become in many countries and regions, economic powerhouses.Construction project is housing planning, survey, design, construction of the floorboard. Purpose is for human life and production provide places.Houses will be like a man, it's like a man's life planning environment is responsible by the planners, Its layout and artistic processing, corresponding to the body shape looks and temperament, is responsible by the architect, Its structure is like a person's bones and life expectancy, the structural engineer is responsible, Its water, heating ventilation and electrical facilities such as the human organ and the nerve, is by the equipment engineer is responsible for. Also like nature intact shaped like people, in the city I district planning based on build houses, and is the construction unit, reconnaissance unit, design unit of various design engineers and construction units comprehensive coordination and cooperation process.After all, but is structural stress body reaction force and the internal stress and how external force balance. Building to tackle, also must solve the problem is mechanical problems. We have to solve the problem of discipline called architectural mechanics. Architectural mechanics have can be divided into: statics, material mechanics and structural mechanics three mechanical system. Architectural mechanics is discussion and research building structure and component in load and other factors affecting the working condition of, also is the building of intensity, stiffness and stability. In load, bear load and load of structure and component can cause the surrounding objects in their function, and the object itself by the load effect and deformation, and there is the possibility of damage, but the structure itself has certain resistance to deformation and destruction of competence, and the bearing capacity of the structure size is and component of materials, cross section, and the structural properties of geometry size, working conditions and structure circumstance relevant. While these relationships can be improved by mechanics formula solved through calculation.Building materials in building and has a pivotal role. Building material is with human society productivity and science and technologyimproves gradually developed. In ancient times, the human lives, the line USES is the rocks andTrees. The 4th century BC, 12 ~ has created a tile and brick, humans are only useful synthetic materials made of housing. The 17th century had cast iron and ShouTie later, until the eighteenth century had Portland cement, just make later reinforced concrete engineering get vigorous development. Now all sorts of high-strength structural materials, new decoration materials and waterproof material development, criterion and 20th century since mid organic polymer materials in civil engineering are closely related to the widely application. In all materials, the most main and most popular is steel, concrete, lumber, masonry. In recent years, by using two kinds of material advantage, will make them together, the combination of structure was developed. Now, architecture, engineering quality fit and unfit quality usually adopted materials quality, performance and using reasonable or not have direct connection, in meet the same technical indicators and quality requirements, under the precondition of choice of different material is different, use method of engineering cost has direct impact.In construction process, building construction is and architectural mechanics, building materials also important links. Construction is to the mind of the designer, intention and idea into realistic process, from the ancient hole JuChao place to now skyscrapers, from rural to urban country road elevated road all need through "construction" means. A construction project, including many jobs such as dredging engineering, deep foundation pit bracing engineering, foundation engineering, reinforced concrete structure engineering, structural lifting project, waterproofing, decorate projects, each type of project has its own rules, all need according to different construction object and construction environment conditions using relevant construction technology, in work-site.whenever while, need and the relevant hydropower and other equipment composition of a whole, each project between reasonable organizing and coordination, better play investment benefit. Civil engineering construction in the benefit, while also issued by the state in strict accordance with the relevant construction technology standard, thus further enhance China's construction level to ensure construction quality, reduce the cost for the project.Any building built on the surface of the earth all strata, building weight eventually to stratum, have to bear. Formation Support building the rocks were referred to as foundation, and the buildings on the ground and under the upper structure of self-respect and liable to load transfer to the foundation of components or component called foundation. Foundation, and the foundation and the superstructure is a building of three inseparable part. According to the function is different, but in load, under the action of them are related to each other, is theinteraction of the whole. Foundation can be divided into natural foundation and artificial foundation, basic according to the buried depth is divided into deep foundation and shallow foundation. , foundation and foundation is the guarantee of the quality of the buildings and normal use close button, where buildings foundation in building under loads of both must maintain overall stability and if the settlement of foundation produce in building scope permitted inside, and foundation itself should have sufficient strength, stiffness and durability, also consider repair methods and the necessary foundation soil retaining retaining water and relevant measures. [3]As people living standard rise ceaselessly, the people to their place of building space has become not only from the number, and put forward higher requirement from quality are put car higher demands that the environment is beautiful, have certain comfort. This needs to decorate a building to be necessary. If architecture major engineering constitutes the skeleton of the building, then after adornment building has become the flesh-and-blood organism, final with rich, perfect appearance in people's in front, the best architecture should fully embody all sorts of adornment material related properties, with existing construction technology, the most effective gimmick, to achieve conception must express effect. Building outfit fix to consider the architectural space use requirement, protect the subject institutions from damage, give a person with beautifulenjoying, satisfy the requirements of fire evacuation, decorative materials and scheme of rationality, construction technology and economic feasibility, etc. Housing construction development and at the same time, like housing construction as affecting people life of roads, Bridges, tunnels has made great progress.In general civil engineering is one of the oldest subjects, it has made great achievements, the future of the civil engineering will occupy in people's life more important position. The environment worsening population increase, people to fight for survival, to strive for a more comfortable living environment, and will pay more attention to civil engineering. In the near future, some major projects extimated to build, insert roller skyscrapers, across the oceanBridges, more convenient traffic would not dream. The development of science and technology, and the earth is deteriorating environment will be prompted civil engineering to aerospace and Marine development, provide mankind broader space of living. In recent years, engineering materials mainly is reinforced concrete, lumber and brick materials, in the future, the traditional materials will be improved, more suitable for some new building materials market, especially the chemistry materials will promote the construction of towards a higher point. Meanwhile, design method of precision, design work of automation, information and intelligent technology of introducing, will be people have a morecomfortable living environment. The word, and the development of the theory and new materials, the emergence of the application of computer, high-tech introduction to wait to will make civil engineering have a new leap.This is a door needs calm and a great deal of patience and attentive professional. Because hundreds of thousands, even hundreds of thousands of lines to building each place structure clearly reflected. Without a gentle state of mind, do what thing just floating on the surface, to any a building structure, to be engaged in business and could not have had a clear, accurate and profound understanding of, the nature is no good. In this business, probably not burn the midnight oil of courage, not to reach the goal of spirit not to give up, will only be companies eliminated.This is a responsible and caring industry. Should have a single responsible heart - I one's life in my hand, thousands of life in my hand. Since the civil, should choose dependably shoulder the responsibility.Finally, this is a constant pursuit of perfect industry. Pyramid, spectacular now: The Great Wall, the majestic... But if no generations of the pursuit of today, we may also use the sort of the oldest way to build this same architecture. Design a building structure is numerous, but this is all experienced centuries of clarification, through continuous accumulation, keep improving, innovation obtained. And such pursuit, not confined in the past. Just think, if the design of a building can be like calculation one plus one equals two as simple and easy to grasp, that was not for what? Therefore, a civil engineer is in constant of in formation. One of the most simple structure, the least cost, the biggest function. Choose civil, choosing a steadfast diligence, innovation, pursuit of perfect path.Reference:[1] LuoFuWu editor. Civil engineering (professional). Introduction to wuhan. Wuhan university of technology press. 2007[2] WangFuChuan, palace rice expensive editor. Construction engineering materials. Beijing. Science and technology literature press. 2002[3] jiang see whales, zhiming editor. Civil engineering introduction of higher education press. Beijing.. 1992土木工程概论 [译文]摘要:土木工程是个庞大的学科,但最主要的是建筑,建筑无论是在中国还是在国外,都有着悠久的历史,长期的发展历程。
土木工程专业英语课文翻译考试必备
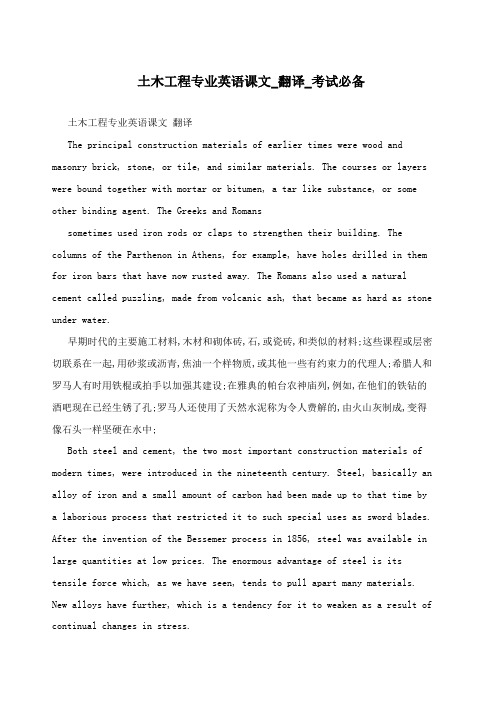
土木工程专业英语课文_翻译_考试必备土木工程专业英语课文翻译The principal construction materials of earlier times were wood and masonry brick, stone, or tile, and similar materials. The courses or layers were bound together with mortar or bitumen, a tar like substance, or some other binding agent. The Greeks and Romanssometimes used iron rods or claps to strengthen their building. The columns of the Parthenon in Athens, for example, have holes drilled in them for iron bars that have now rusted away. The Romans also used a natural cement called puzzling, made from volcanic ash, that became as hard as stone under water.早期时代的主要施工材料,木材和砌体砖,石,或瓷砖,和类似的材料;这些课程或层密切联系在一起,用砂浆或沥青,焦油一个样物质,或其他一些有约束力的代理人;希腊人和罗马人有时用铁棍或拍手以加强其建设;在雅典的帕台农神庙列,例如,在他们的铁钻的酒吧现在已经生锈了孔;罗马人还使用了天然水泥称为令人费解的,由火山灰制成,变得像石头一样坚硬在水中;Both steel and cement, the two most important construction materials of modern times, were introduced in the nineteenth century. Steel, basically an alloy of iron and a small amount of carbon had been made up to that time by a laborious process that restricted it to such special uses as sword blades. After the invention of the Bessemer process in 1856, steel was available in large quantities at low prices. The enormous advantage of steel is its tensile force which, as we have seen, tends to pull apart many materials. New alloys have further, which is a tendency for it to weaken as a result of continual changes in stress.钢铁和水泥,两个最重要的现代建筑材料,介绍了在十九世纪;钢,铁,基本上是少量的碳合金已作出了这一由一个艰苦的过程,限制它的刀刃等特殊用途的时间;后在1856年发明贝塞麦过程,钢在低价格大批量供货;钢铁的巨大优势是它的拉伸力,正如我们所看到的,往往会拉开许多材料;新合金进一步,这是一个趋势,它削弱了在压力不断变化的结果;Modern cement, called Portland cement, was invented in 1824. It is a mixture of limestone and clay, which is heated and then ground into a power. It is mixed at or near the construction site with sand, aggregate small stones, crushed rock, or gravel, and water to make concrete. Different proportions of the ingredients produce concrete with different strength and weight. Concrete is very versatile; it can be poured, pumped, or even sprayed into all kinds of shapes. And whereas steel has great tensile strength, concrete has great strength under compression. Thus, the two substances complement each other. 现代水泥,称为硅酸盐水泥,发明于1824年;它是石灰石和粘土的混合物,被加热,然后进入电源地;它是混合达到或接近沙施工现场,聚集的小石头,碎石,或石子和水,使混凝土;不同比例的成分产生不同强度和重量混凝土;混凝土是非常灵活,它可浇,泵浦,或连成各种形状喷洒;和鉴于钢具有很大的拉伸强度,混凝土受压的伟大力量;因此,这两种物质是相辅相成的;They also complement each other in another way: they have almost the same rate of contraction and expansion. They therefore can work together in situations where both compression and tension are factors. Steel rods are embedded in concrete to make reinforced concrete in concrete beams or structures where tensions will develop. Concrete and steel also form such a strong bond the force that unites them that the steel cannot slip within theconcrete. Still another advantage is that steel does not rust in concrete. Acid corrodessteel, whereas concrete has an alkaline chemical reaction, the oppositeof acid.他们还以另一种方式补充对方:他们几乎在同样的速度收缩和扩张;因此,他们可以一起工作的情况下压缩和紧张的因素;钢条是嵌在混凝土,使钢筋混凝土结构中混凝土梁或地方的紧张局势会发展;混凝土和钢也形成如此强烈的纽带团结的力量他们的钢材,不滑内的混凝土;还有一个好处是,不生锈的钢混凝土;酸腐蚀钢,而混凝土的碱性化学反应,酸相反;The adoption of structural steel and reinforced concrete caused major changes in traditional construction practices. It was no longer necessary to use thick walls of stone or brick for multistory buildings, and it became much simpler to build fire-resistant floors. Both these changes served to reduce the cost of construction. It also became possible to erect buildings with greater heights and longer spans.结构钢和钢筋混凝土建筑采用传统的做法造成了重大变化;它不再需要使用的石块或砖头厚的多层建筑物的墙壁,成为更简单,建立防火地板;这些变化都有助于降低建设成本;它也成为可能有更大的直立高度和时间跨度的建筑;Since the weight of modern structures is carried by the steel or concrete frame, the walls do not support the building. They have become curtain walls, which keep out the weather and let in light. In the earlier steel orconcrete frame building, the curtain walls were generally made of masonry; they had the solid look of bearing walls. Today, however, curtain walls are often made of lightweight materials such as glass, aluminum, or plastic, in various combinations. 由于现代结构重量是由钢或混凝土框架进行,墙壁不支持建设;他们已成为玻璃幕墙,它保持了天气和光线让;在早期的钢或混凝土框架结构,玻璃幕墙,一般由砖石,他们有坚实的承重墙看看;然而,今天,玻璃幕墙往往是由诸如玻璃,铝,塑料或轻质材料,在各种组合;Another advance in steel construction is the method of fastening together the beams. For many years the standard method was riveting. A rivet is abolt with a head that looks like a blunt screw without threads. It is heated, placed in holes through the pieces of steel, and a second head is formed at the other end by hammering it to hold it in place. Riveting has now largely been replaced by welding, thejoining together of pieces of steel by melting a steel material between them under high heat.钢结构建筑的另一个进步是梁紧固在一起的方法;多年来,标准方法是铆;铆钉是一个头,像一个没有线程看起来钝螺丝螺栓;它被加热时,通过放置在洞的钢件,第二头在另一端形成的锤击它举行到位;铆接现在很大程度上是由焊接取代,加入钢件在一起融化在高温下它们之间的钢铁材料;Presstressed concrete is an improved form of reinforcement. Steel rodsare bent into the shapes to give them the necessary degree of tensile strengths. They are then used to priestess concrete, usually by one of two different methods. The first is to leave channels in a concrete beam that correspond to the shapes of the steel rods. When the rods are run throughthe channels, they are then bonded to the concrete by filling the channels with grout, a thin mortar or binding agent. In the other and more common method, the priestesses steel rods are placed in the lower part of a formthat corresponds to the shape of the finished structure, and the concrete is poured around them. Priestess’s concrete uses less steel and less concrete. Because it is a highly desirable material.预应力钢筋混凝土是一种改进形式;棒钢弯曲成的形状,给他们一定程度的拉伸强度;然后他们用女祭司混凝土,由两种不同的方法之一,通常;首先是留在渠道混凝土梁对应于钢铁棒的形状;当棒是通过渠道来说,他们是那么粘在混凝土充填灌浆,薄砂浆或结合剂的渠道;在其他更常见的方法,女祭司钢棒放置在一个表格对应的成品下部结构形状,和他们周围的混凝土浇;女祭司的具体使用较少的钢铁和混凝土少;因为它是一个非常可取的材料;Presstressed concrete has made it possible to develop buildings with unusual shapes, like some of the modern, sports arenas, with large spaces unbroken by any obstructing supports. The uses for this relatively new structural method are constantly being developed. 预应力混凝土使人们有可能发展不寻常的形状的建筑物,如现代,体育场一些大空间的任何阻挠支持不间断;在使用这种相对较新的构造方法正在不断发展;。
土木工程毕业设计外文翻译原文+翻译

The bridge crack produced the reason to simply analyseIn recent years, the traffic capital construction of our province gets swift and violent development, all parts have built a large number of concrete bridges. In the course of building and using in the bridge, relevant to influence project quality lead of common occurrence report that bridge collapse even because the crack appears The concrete can be said to " often have illness coming on " while fracturing and " frequently-occurring disease ", often perplex bridge engineers and technicians. In fact , if take certain design and construction measure, a lot of cracks can be overcome and controlled. For strengthen understanding of concrete bridge crack further, is it prevent project from endanger larger crack to try one's best, this text make an more overall analysis , summary to concrete kind and reason of production , bridge of crack as much as possible, in order to design , construct and find out the feasible method which control the crack , get the result of taking precautions against Yu WeiRan.Concrete bridge crack kind, origin cause of formation In fact, the origin cause of formation of the concrete structure crack is complicated and various, even many kinds of factors influence each other , but every crack has its one or several kinds of main reasons produced . The kind of the concrete bridge crack, on its reason to produce, can roughly divide several kinds as follows :(1) load the crack caused Concrete in routine quiet .Is it load to move and crack that produce claim to load the crack under the times of stress bridge, summing up has direct stress cracks , two kinds stress crack onces mainly. Direct stress crack refer to outside load direct crack that stress produce that cause. The reason why the crack produces is as follows, 1, Design the stage of calculating , does not calculate or leaks and calculates partly while calculating in structure; Calculate the model is unreasonable; The structure is supposed and accorded with by strength actually by strength ; Load and calculate or leak and calculate few; Internal force and matching the mistake in computation of muscle; Safety coefficient of structure is not enough. Do not consider the possibility that construct at the time of the structural design; It is insufficientto design the section; It is simply little and assigning the mistake for reinforcing bar to set up; Structure rigidity is insufficient; Construct and deal with improperly; The design drawing can not be explained clearly etc.. 2, Construction stage, does not pile up and construct the machines , material limiting ; Is it prefabricate structure structure receive strength characteristic , stand up , is it hang , transport , install to get up at will to understand; Construct not according to the design drawing, alter the construction order of the structure without authorization , change the structure and receive the strength mode; Do not do the tired intensity checking computations under machine vibration and wait to the structure . 3, Using stage, the heavy-duty vehicle which goes beyond the design load passes the bridge; Receive the contact , striking of the vehicle , shipping; Strong wind , heavy snow , earthquake happen , explode etc.. Stress crack once means the stress of secondary caused by loading outside produces the crack. The reason why the crack produces is as follows, 1, In design outside load function , because actual working state and routine , structure of thing calculate have discrepancy or is it consider to calculate, thus cause stress once to cause the structure to fracture in some position. Two is it join bridge arch foot is it is it assign " X " shape reinforcing bar , cut down this place way , section of size design and cut with scissors at the same time to adopt often to design to cut with scissors, theory calculate place this can store curved square in , but reality should is it can resist curved still to cut with scissors, so that present the crack and cause the reinforcing bar corrosion. 2, Bridge structure is it dig trough , turn on hole , set up ox leg ,etc. to need often, difficult to use a accurate one diagrammatic to is it is it calculate to imitate to go on in calculating in routine, set up and receive the strength reinforcing bar in general foundation experience. Studies have shown , after being dug the hole by the strength component , it will produce the diffraction phenomenon that strength flows, intensive near the hole in a utensil, produced the enormous stress to concentrate. In long to step prestressing force of the continuous roof beam , often block the steel bunch according to the needs of section internal force in stepping, set up the anchor head, but can often see the crack in the anchor firm section adjacent place. So if deal with improper, in corner or component form sudden change office , block place to be easy to appear crack strengthreinforcing bar of structure the. In the actual project, stress crack once produced the most common reason which loads the crack. Stress crack once belong to one more piece of nature of drawing , splitting off , shearing. Stress crack once is loaded and caused, only seldom calculate according to the routine too, but with modern to calculate constant perfection of means, times of stress crack to can accomplish reasonable checking computations too. For example to such stresses 2 times of producing as prestressing force , creeping ,etc., department's finite element procedure calculates levels pole correctly now, but more difficult 40 years ago. In the design, should pay attention to avoiding structure sudden change (or section sudden change), when it is unable to avoid , should do part deal with , corner for instance, make round horn , sudden change office make into the gradation zone transition, is it is it mix muscle to construct to strengthen at the same time, corner mix again oblique to reinforcing bar , as to large hole in a utensil can set up protecting in the perimeter at the terms of having angle steel. Load the crack characteristic in accordance with loading differently and presenting different characteristics differently. The crack appear person who draw more, the cutting area or the serious position of vibration. Must point out , is it get up cover or have along keep into short crack of direction to appear person who press, often the structure reaches the sign of bearing the weight of strength limit, it is an omen that the structure is destroyed, its reason is often that sectional size is partial and small. Receive the strength way differently according to the structure, the crack characteristic produced is as follows: 1, The centre is drawn. The crack runs through the component cross section , the interval is equal on the whole , and is perpendicular to receiving the strength direction. While adopting the whorl reinforcing bar , lie in the second-class crack near the reinforcing bar between the cracks. 2, The centre is pressed. It is parallel on the short and dense parallel crack which receive the strength direction to appear along the component. 3, Receive curved. Most near the large section from border is it appear and draw into direction vertical crack to begin person who draw curved square, and develop toward neutralization axle gradually. While adopting the whorl reinforcing bar , can see shorter second-class crack among the cracks. When the structure matches muscles less, there are few but wide cracks, fragility destruction may take place in thestructure 4, Pressed big and partial. Heavy to press and mix person who draw muscle a less one light to pigeonhole into the component while being partial while being partial, similar to receiving the curved component. 5, Pressed small and partial. Small to press and mix person who draw muscle a more one heavy to pigeonhole into the component while being partial while being partial, similar to the centre and pressed the component. 6, Cut. Press obliquly when the hoop muscle is too dense and destroy, the oblique crack which is greater than 45?? direction appears along the belly of roof beam end; Is it is it is it destroy to press to cut to happen when the hoop muscle is proper, underpart is it invite 45?? direction parallel oblique crack each other to appear along roof beam end. 7, Sprained. Component one side belly appear many direction oblique crack, 45?? of treaty, first, and to launch with spiral direction being adjoint. 8, Washed and cut. 4 side is it invite 45?? direction inclined plane draw and split to take place along column cap board, form the tangent plane of washing. 9, Some and is pressed. Some to appear person who press direction roughly parallel large short cracks with pressure.(2) crack caused in temperature changeThe concrete has nature of expanding with heat and contract with cold, look on as the external environment condition or the structure temperature changes, concrete take place out of shape, if out of shape to restrain from, produce the stress in the structure, produce the temperature crack promptly when exceeding concrete tensile strength in stress. In some being heavy to step foot-path among the bridge , temperature stress can is it go beyond living year stress even to reach. The temperature crack distinguishes the main characteristic of other cracks will be varied with temperature and expanded or closed up. The main factor is as follows, to cause temperature and change 1, Annual difference in temperature. Temperature is changing constantly in four seasons in one year, but change relatively slowly, the impact on structure of the bridge is mainly the vertical displacement which causes the bridge, can prop up seat move or set up flexible mound ,etc. not to construct measure coordinate , through bridge floor expansion joint generally, can cause temperature crack only when the displacement of the structure is limited, for example arched bridge , just bridge etc. The annual difference in temperature of our country generally changes therange with the conduct of the average temperature in the moon of January and July. Considering the creep characteristic of the concrete, the elastic mould amount of concrete should be considered rolling over and reducing when the internal force of the annual difference in temperature is calculated. 2, Rizhao. After being tanned by the sun by the sun to the side of bridge panel , the girder or the pier, temperature is obviously higher than other position, the temperature gradient is presented and distributed by the line shape . Because of restrain oneself function, cause part draw stress to be relatively heavy, the crack appears. Rizhao and following to is it cause structure common reason most , temperature of crack to lower the temperature suddenly 3, Lower the temperature suddenly. Fall heavy rain , cold air attack , sunset ,etc. can cause structure surface temperature suddenly dropped suddenly, but because inside temperature change relatively slow producing temperature gradient. Rizhao and lower the temperature internal force can adopt design specification or consult real bridge materials go on when calculating suddenly, concrete elastic mould amount does not consider converting into and reducing 4, Heat of hydration. Appear in the course of constructing, the large volume concrete (thickness exceeds 2. 0), after building because cement water send out heat, cause inside very much high temperature, the internal and external difference in temperature is too large, cause the surface to appear in the crack. Should according to actual conditions in constructing, is it choose heat of hydration low cement variety to try one's best, limit cement unit's consumption, reduce the aggregate and enter the temperature of the mould , reduce the internal and external difference in temperature, and lower the temperature slowly , can adopt the circulation cooling system to carry on the inside to dispel the heat in case of necessity, or adopt the thin layer and build it in succession in order to accelerate dispelling the heat. 5, The construction measure is improper at the time of steam maintenance or the winter construction , the concrete is sudden and cold and sudden and hot, internal and external temperature is uneven , apt to appear in the crack. 6, Prefabricate T roof beam horizontal baffle when the installation , prop up seat bury stencil plate with transfer flat stencil plate when welding in advance, if weld measure to be improper, iron pieces of nearby concrete easy to is it fracture to burn. Adopt electric heat piece draw law piece draw prestressing force at the component ,prestressing force steel temperature can rise to 350 degrees Centigrade , the concrete component is apt to fracture. Experimental study indicates , are caused the intensity of concrete that the high temperature burns to obviously reduce with rising of temperature by such reasons as the fire ,etc., glueing forming the decline thereupon of strength of reinforcing bar and concrete, tensile strength drop by 50% after concrete temperature reaches 300 degrees Centigrade, compression strength drops by 60%, glueing the strength of forming to drop by 80% of only round reinforcing bar and concrete; Because heat, concrete body dissociate ink evaporate and can produce and shrink sharply in a large amount(3) shrink the crack causedIn the actual project, it is the most common because concrete shrinks the crack caused. Shrink kind in concrete, plasticity shrink is it it shrinks (is it contract to do ) to be the main reason that the volume of concrete out of shape happens to shrink, shrink spontaneously in addition and the char shrink. Plasticity shrink. About 4 hours after it is built that in the course of constructing , concrete happens, the cement water response is fierce at this moment, the strand takes shape gradually, secrete water and moisture to evaporate sharply, the concrete desiccates and shrinks, it is at the same time conduct oneself with dignity not sinking because aggregate,so when harden concrete yet,it call plasticity shrink. The plasticity shrink producing amount grade is very big, can be up to about 1%. If stopped by the reinforcing bar while the aggregate sinks, form the crack along the reinforcing bar direction. If web , roof beam of T and roof beam of case and carry baseplate hand over office in component vertical to become sectional place, because sink too really to superficial obeying the web direction crack will happen evenly before hardenning. For reducing concrete plasticity shrink,it should control by water dust when being construct than,last long-time mixing, unloading should not too quick, is it is it take closely knit to smash to shake, vertical to become sectional place should divide layer build. Shrink and shrink (do and contract). After the concrete is formed hard , as the top layer moisture is evaporated progressively , the humidity is reduced progressively , the volume of concrete is reduced, is called and shrunk to shrink (do and contract). Because concrete top layermoisture loss soon, it is slow for inside to lose, produce surface shrink heavy , inside shrink a light one even to shrink, it is out of shape to restrain from by the inside concrete for surface to shrink, cause the surface concrete to bear pulling force, when the surface concrete bears pulling force to exceed its tensile strength, produce and shrink the crack. The concrete hardens after-contraction to just shrink and shrink mainly .Such as mix muscle rate heavy component (exceed 3% ), between reinforcing bar and more obvious restraints relatively that concrete shrink, the concrete surface is apt to appear in the full of cracks crackle. Shrink spontaneously. Spontaneous to it shrinks to be concrete in the course of hardenning , cement and water take place ink react, the shrink with have nothing to do by external humidity, and can positive (whether shrink, such as ordinary portland cement concrete), can negative too (whether expand, such as concrete, concrete of slag cement and cement of fly ash). The char shrinks. Between carbon dioxide and hyrate of cement of atmosphere take place out of shape shrink that chemical reaction cause. The char shrinks and could happen only about 50% of humidity, and accelerate with increase of the density of the carbon dioxide. The char shrinks and seldom calculates . The characteristic that the concrete shrinks the crack is that the majority belongs to the surface crack, the crack is relatively detailed in width , and criss-cross, become the full of cracks form , the form does not have any law . Studies have shown , influence concrete shrink main factor of crack as follows, 1, Variety of cement , grade and consumption. Slag cement , quick-hardening cement , low-heat cement concrete contractivity are relatively high, ordinary cement , volcanic ash cement , alumina cement concrete contractivity are relatively low. Cement grade low in addition, unit volume consumption heavy rubing detailed degree heavy, then the concrete shrinks the more greatly, and shrink time is the longer. For example, in order to improve the intensity of the concrete , often adopt and increase the cement consumption method by force while constructing, the result shrinks the stress to obviously strengthen . 2, Variety of aggregate. Such absorbing water rates as the quartz , limestone , cloud rock , granite , feldspar ,etc. are smaller, contractivity is relatively low in the aggregate; And such absorbing water rates as the sandstone , slate , angle amphibolite ,etc. are greater, contractivity is relatively high. Aggregate grains of foot-path heavy to shrink light inaddition, water content big to shrink the larger. 3, Water gray than. The heavier water consumption is, the higher water and dust are, the concrete shrinks the more greatly. 4, Mix the pharmaceutical outside. It is the better to mix pharmaceutical water-retaining property outside, then the concrete shrinks the smaller. 5, Maintain the method . Water that good maintenance can accelerate the concrete reacts, obtain the intensity of higher concrete. Keep humidity high , low maintaining time to be the longer temperature when maintaining, then the concrete shrinks the smaller. Steam maintain way than maintain way concrete is it take light to shrink naturall. 6, External environment. The humidity is little, the air drying , temperature are high, the wind speed is large in the atmosphere, then the concrete moisture is evaporated fast, the concrete shrinks the faster. 7, Shake and smash the way and time. Machinery shake way of smashing than make firm by ramming or tamping way concrete contractivity take little by hand. Shaking should determine according to mechanical performance to smash time , are generally suitable for 55s / time. It is too short, shake and can not smash closely knit , it is insufficient or not even in intensity to form the concrete; It is too long, cause and divide storey, thick aggregate sinks to the ground floor, the upper strata that the detailed aggregate stays, the intensity is not even , the upper strata incident shrink the crack. And shrink the crack caused to temperature, worthy of constructing the reinforcing bar againing can obviously improve the resisting the splitting of concrete , structure of especially thin wall (thick 200cm of wall ). Mix muscle should is it adopt light diameter reinforcing bar (8 |? construct 14 |? ) to have priority , little interval assign (whether @ 10 construct @ 15cm ) on constructing, the whole section is it mix muscle to be rate unsuitable to be lower than 0 to construct. 3%, can generally adopt 0 . 3%~0. 5%.(4), crack that causes out of shape of plinth of the groundBecause foundation vertical to even to subside or horizontal direction displacement, make the structure produce the additional stress, go beyond resisting the ability of drawing of concrete structure, cause the structure to fracture. The even main reason that subside of the foundation is as follows, 1, Reconnoitres the precision and is not enough for , test the materials inaccuratly in geology. Designing, constructing without fully grasping the geological situation, this is the main reason that cause the ground not to subside evenly .Such as hills area or bridge, district of mountain ridge,, hole interval to be too far when reconnoitring, and ground rise and fall big the rock, reconnoitring the report can't fully reflect the real geological situation . 2, The geological difference of the ground is too large. Building it in the bridge of the valley of the ditch of mountain area, geology of the stream place and place on the hillside change larger, even there are weak grounds in the stream, because the soil of the ground does not causes and does not subside evenly with the compressing. 3, The structure loads the difference too big. Under the unanimous terms, when every foundation too heavy to load difference in geological situation, may cause evenly to subside, for example high to fill out soil case shape in the middle part of the culvert than to is it take heavy to load both sides, to subside soon heavy than both sides middle part, case is it might fracture to contain 4, The difference of basic type of structure is great. Unite it in the bridge the samly , mix and use and does not expand the foundation and a foundation with the foundation, or adopt a foundation when a foot-path or a long difference is great at the same time , or adopt the foundation of expanding when basis elevation is widely different at the same time , may cause the ground not to subside evenly too 5, Foundation built by stages. In the newly-built bridge near the foundation of original bridge, if the half a bridge about expressway built by stages, the newly-built bridge loads or the foundation causes the soil of the ground to consolidate again while dealing with, may cause and subside the foundation of original bridge greatly 6, The ground is frozen bloatedly. The ground soil of higher moisture content on terms that lower than zero degree expands because of being icy; Once temperature goes up , the frozen soil is melted, the setting of ground. So the ground is icy or melts causes and does not subside evenly . 7, Bridge foundation put on body, cave with stalactites and stalagmites, activity fault,etc. of coming down at the bad geology, may cause and does not subside evenly . 8, After the bridge is built up , the condition change of original ground . After most natural grounds and artificial grounds are soaked with water, especially usually fill out such soil of special ground as the soil , loess , expanding in the land ,etc., soil body intensity meet water drop, compress out of shape to strengthen. In the soft soil ground , season causes the water table to drop to draw water or arid artificially, the ground soil layer consolidates and sinks again,reduce the buoyancy on the foundation at the same time , shouldering the obstruction of rubing to increase, the foundation is carried on one's shoulder or back and strengthened .Some bridge foundation is it put too shallow to bury, erode , is it dig to wash flood, the foundation might be moved. Ground load change of terms, bridge nearby is it is it abolish square , grit ,etc. in a large amount to put to pile with cave in , landslide ,etc. reason for instance, it is out of shape that the bridge location range soil layer may be compressed again. So, the condition of original ground change while using may cause and does not subside evenly Produce the structure thing of horizontal thrust to arched bridge ,etc., it is the main reason that horizontal displacement crack emerges to destroy the original geological condition when to that it is unreasonable to grasp incompletely , design and construct in the geological situation.桥梁裂缝产生原因浅析近年来,我省交通基础建设得到迅猛发展,各地建立了大量的混凝土桥梁。
土木工程英文文献及翻译
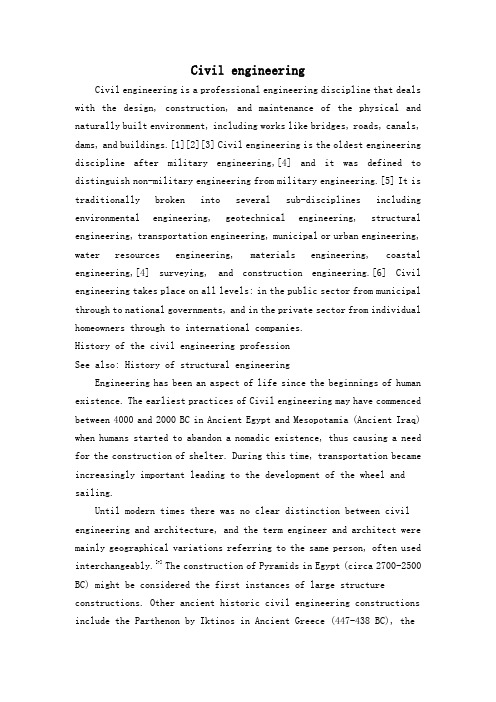
Civil engineeringCivil engineering is a professional engineering discipline that deals with the design, construction, and maintenance of the physical and naturally built environment, including works like bridges, roads, canals, dams, and buildings.[1][2][3] Civil engineering is the oldest engineering discipline after military engineering,[4] and it was defined to distinguish non-military engineering from military engineering.[5] It is traditionally broken into several sub-disciplines including environmental engineering, geotechnical engineering, structural engineering, transportation engineering, municipal or urban engineering, water resources engineering, materials engineering, coastal engineering,[4] surveying, and construction engineering.[6] Civil engineering takes place on all levels: in the public sector from municipal through to national governments, and in the private sector from individual homeowners through to international companies.History of the civil engineering professionSee also: History of structural engineeringEngineering has been an aspect of life since the beginnings of human existence. The earliest practices of Civil engineering may have commenced between 4000 and 2000 BC in Ancient Egypt and Mesopotamia (Ancient Iraq) when humans started to abandon a nomadic existence, thus causing a need for the construction of shelter. During this time, transportation became increasingly important leading to the development of the wheel and sailing.Until modern times there was no clear distinction between civil engineering and architecture, and the term engineer and architect were mainly geographical variations referring to the same person, often used interchangeably.[7]The construction of Pyramids in Egypt (circa 2700-2500 BC) might be considered the first instances of large structure constructions. Other ancient historic civil engineering constructions include the Parthenon by Iktinos in Ancient Greece (447-438 BC), theAppian Way by Roman engineers (c. 312 BC), the Great Wall of China by General Meng T'ien under orders from Ch'in Emperor Shih Huang Ti (c. 220 BC)[6] and the stupas constructed in ancient Sri Lanka like the Jetavanaramaya and the extensive irrigation works in Anuradhapura. The Romans developed civil structures throughout their empire, including especially aqueducts, insulae, harbours, bridges, dams and roads.In the 18th century, the term civil engineering was coined to incorporate all things civilian as opposed to military engineering.[5]The first self-proclaimed civil engineer was John Smeaton who constructed the Eddystone Lighthouse.[4][6]In 1771 Smeaton and some of his colleagues formed the Smeatonian Society of Civil Engineers, a group of leaders of the profession who met informally over dinner. Though there was evidence of some technical meetings, it was little more than a social society.In 1818 the Institution of Civil Engineers was founded in London, and in 1820 the eminent engineer Thomas Telford became its first president. The institution received a Royal Charter in 1828, formally recognising civil engineering as a profession. Its charter defined civil engineering as:the art of directing the great sources of power in nature for the use and convenience of man, as the means of production and of traffic in states, both for external and internal trade, as applied in the construction of roads, bridges, aqueducts, canals, river navigation and docks for internal intercourse and exchange, and in the construction of ports, harbours, moles, breakwaters and lighthouses, and in the art of navigation by artificial power for the purposes of commerce, and in the construction and application of machinery, and in the drainage of cities and towns.[8] The first private college to teach Civil Engineering in the United States was Norwich University founded in 1819 by Captain Alden Partridge.[9] The first degree in Civil Engineering in the United States was awarded by Rensselaer Polytechnic Institute in 1835.[10] The first such degree to be awarded to a woman was granted by Cornell University to Nora Stanton Blatchin 1905.History of civil engineeringCivil engineering is the application of physical and scientific principles, and its history is intricately linked to advances in understanding of physics and mathematics throughout history. Because civil engineering is a wide ranging profession, including several separate specialized sub-disciplines, its history is linked to knowledge of structures, materials science, geography, geology, soils, hydrology, environment, mechanics and other fields.Throughout ancient and medieval history most architectural design and construction was carried out by artisans, such as stone masons and carpenters, rising to the role of master builder. Knowledge was retained in guilds and seldom supplanted by advances. Structures, roads and infrastructure that existed were repetitive, and increases in scale were incremental.[12]One of the earliest examples of a scientific approach to physical and mathematical problems applicable to civil engineering is the work of Archimedes in the 3rd century BC, including Archimedes Principle, which underpins our understanding of buoyancy, and practical solutions such as Archimedes' screw. Brahmagupta, an Indian mathematician, used arithmetic in the 7th century AD, based on Hindu-Arabic numerals, for excavation (volume) computations.[13]Civil engineers typically possess an academic degree with a major in civil engineering. The length of study for such a degree is usually three to five years and the completed degree is usually designated as a Bachelor of Engineering, though some universities designate the degree as a Bachelor of Science. The degree generally includes units covering physics, mathematics, project management, design and specific topics in civil engineering. Initially such topics cover most, if not all, of thesub-disciplines of civil engineering. Students then choose to specialize in one or more sub-disciplines towards the end of the degree.[14]While anUndergraduate (BEng/BSc) Degree will normally provide successful students with industry accredited qualification, some universities offer postgraduate engineering awards (MEng/MSc) which allow students to further specialize in their particular area of interest within engineering.[15]In most countries, a Bachelor's degree in engineering represents the first step towards professional certification and the degree program itself is certified by a professional body. After completing a certified degree program the engineer must satisfy a range of requirements (including work experience and exam requirements) before being certified. Once certified, the engineer is designated the title of Professional Engineer (in the United States, Canada and South Africa), Chartered Engineer (in most Commonwealth countries), Chartered Professional Engineer (in Australia and New Zealand), or European Engineer (in much of the European Union). There are international engineering agreements between relevant professional bodies which are designed to allow engineers to practice across international borders.The advantages of certification vary depending upon location. For example, in the United States and Canada "only a licensed engineer may prepare, sign and seal, and submit engineering plans and drawings to a public authority for approval, or seal engineering work for public and private clients.".[16]This requirement is enforced by state and provincial legislation such as Quebec's Engineers Act.[17]In other countries, no such legislation exists. In Australia, state licensing of engineers is limited to the state of Queensland. Practically all certifying bodies maintain a code of ethics that they expect all members to abide by or risk expulsion.[18] In this way, these organizations play an important role in maintaining ethical standards for the profession. Even in jurisdictions where certification has little or no legal bearing on work, engineers are subject to contract law. In cases where an engineer's work fails he or she may be subject to the tort of negligence and, in extreme cases, thecharge of criminal negligence.[citation needed] An engineer's work must also comply with numerous other rules and regulations such as building codes and legislation pertaining to environmental law.CareersThere is no one typical career path for civil engineers. Most people who graduate with civil engineering degrees start with jobs that require a low level of responsibility, and as the new engineers prove their competence, they are trusted with tasks that have larger consequences and require a higher level of responsibility. However, within each branch of civil engineering career path options vary. In some fields and firms, entry-level engineers are put to work primarily monitoring construction in the field, serving as the "eyes and ears" of senior design engineers; while in other areas, entry-level engineers perform the more routine tasks of analysis or design and interpretation. Experienced engineers generally do more complex analysis or design work, or management of more complex design projects, or management of other engineers, or into specialized consulting, including forensic engineering.In general, civil engineering is concerned with the overall interface of human created fixed projects with the greater world. General civil engineers work closely with surveyors and specialized civil engineers to fit and serve fixed projects within their given site, community and terrain by designing grading, drainage, pavement, water supply, sewer service, electric and communications supply, and land divisions. General engineers spend much of their time visiting project sites, developing community consensus, and preparing construction plans. General civil engineering is also referred to as site engineering, a branch of civil engineering that primarily focuses on converting a tract of land from one usage to another. Civil engineers typically apply the principles of geotechnical engineering, structural engineering, environmental engineering, transportation engineering and construction engineering toresidential, commercial, industrial and public works projects of all sizes and levels of construction翻译:土木工程土木工程是一个专业的工程学科,包括设计,施工和维护与环境的改造,涉及了像桥梁,道路,河渠,堤坝和建筑物工程交易土木工程是最古老的军事工程后,工程学科,它被定义为区分军事工程非军事工程的学科它传统分解成若干子学科包括环境工程,岩土工程,结构工程,交通工程,市或城市工程,水资源工程,材料工程,海岸工程,勘测和施工工程等土木工程的范围涉及所有层次:从市政府到国家,从私人部门到国际公司。
土木工程外文翻译-原文
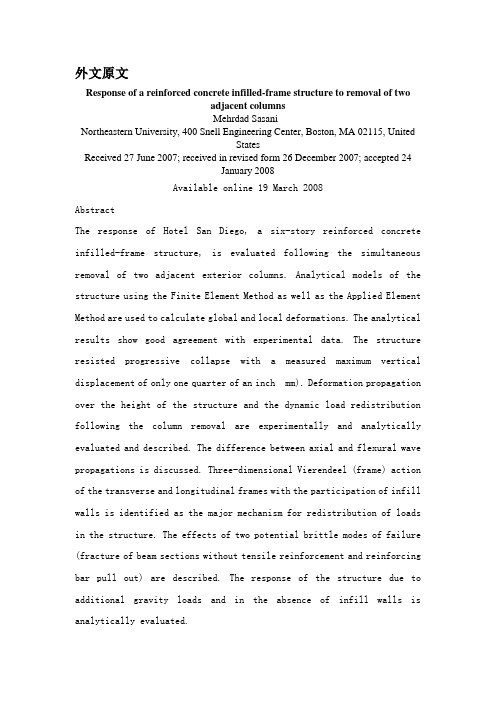
外文原文Response of a reinforced concrete infilled-frame structure to removal of twoadjacent columnsMehrdad Sasani_Northeastern University, 400 Snell Engineering Center, Boston, MA 02115, UnitedStatesReceived 27 June 2007; received in revised form 26 December 2007; accepted 24January 2008Available online 19 March 2008AbstractThe response of Hotel San Diego, a six-story reinforced concrete infilled-frame structure, is evaluated following the simultaneous removal of two adjacent exterior columns. Analytical models of the structure using the Finite Element Method as well as the Applied Element Method are used to calculate global and local deformations. The analytical results show good agreement with experimental data. The structure resisted progressive collapse with a measured maximum vertical displacement of only one quarter of an inch mm). Deformation propagation over the height of the structure and the dynamic load redistribution following the column removal are experimentally and analytically evaluated and described. The difference between axial and flexural wave propagations is discussed. Three-dimensional Vierendeel (frame) action of the transverse and longitudinal frames with the participation of infill walls is identified as the major mechanism for redistribution of loads in the structure. The effects of two potential brittle modes of failure (fracture of beam sections without tensile reinforcement and reinforcing bar pull out) are described. The response of the structure due to additional gravity loads and in the absence of infill walls is analytically evaluated.c 2008 Elsevier Ltd. All rights reserved.Keywords: Progressive collapse; Load redistribution; Load resistance; Dynamic response; Nonlinear analysis; Brittle failure1.IntroductionThe principal scope of specifications is to provide general principles and computational methods in order to verify safet y of structures. The “safety factor ”, which according t o modern trends is independent of the nature and combination of the materials used, can usually be defined as the rati o between the conditions. This ratio is also proportional to the inverse of the probability ( risk ) of failure of th e structure.Failure has to be considered not only as overall collapse o f the structure but also as unserviceability or, according t o a more precise. Common definition. As the reaching of a “limit state ”which causes the construction not to acco mplish the task it was designed for. There are two categori es of limit state :(1)Ultimate limit sate, which corresponds to the highest value of the load-bearing capacity. Examples include local buckli ng or global instability of the structure; failure of some sections and subsequent transformation of the structure intoa mechanism; failure by fatigue; elastic or plastic deformati on or creep that cause a substantial change of the geometry of the structure; and sensitivity of the structure to alte rnating loads, to fire and to explosions.(2)Service limit states, which are functions of the use and durability of the structure. Examples include excessive defo rmations and displacements without instability; early or exces sive cracks; large vibrations; and corrosion.Computational methods used to verify structures with respect to the different safety conditions can be separated into: (1)Deterministic methods, in which the main parameters are co nsidered as nonrandom parameters.(2)Probabilistic methods, in which the main parameters are co nsidered as random parameters.Alternatively, with respect to the different use of factors of safety, computational methods can be separated into:(1)Allowable stress method, in which the stresses computed un der maximum loads are compared with the strength of the mat erial reduced by given safety factors.(2)Limit states method, in which the structure may be propor tioned on the basis of its maximum strength. This strength, as determined by rational analysis, shall not be less than that required to support a factored load equal to the sum of the factored live load and dead load ( ultimate state ).The stresses corresponding to working ( service ) conditions with unfactored live and dead loads are compared with pres cribed values ( service limit state ) . From the four poss ible combinations of the first two and second two methods, we can obtain some useful computational methods. Generally, t wo combinations prevail:(1)deterministic methods, which make use of allowable stresses . (2)Probabilistic methods, which make use of limit states. The main advantage of probabilistic approaches is that, at l east in theory, it is possible to scientifically take into account all random factors of safety, which are then combine d to define the safety factor. probabilistic approaches depend upon :(1) Random distribution of strength of materials with respect to the conditions of fabrication and erection ( scatter of the values of mechanical properties through out the structu re ); (2) Uncertainty of the geometry of the cross-section sand of the structure ( faults and imperfections due to fab rication and erection of the structure );(3) Uncertainty of the predicted live loads and dead loads acting on the structure; (4)Uncertainty related to the approx imation of the computational method used ( deviation of the actual stresses from computed stresses ). Furthermore, proba bilistic theories mean that the allowable risk can be based on several factors, such as :(1) Importance of the construction and gravity of the damage by its failure; (2)Number of human lives which can be thr eatened by this failure; (3)Possibility and/or likelihood of repairing the structure; (4) Predicted life of the structure. All these factors are related to economic and social consi derations such as:(1) Initial cost of the construction;(2) Amortization funds for the duration of the construction;(3) Cost of physical and material damage due to the failure of the construction;(4) Adverse impact on society;(5) Moral and psychological views.The definition of all these parameters, for a given saf ety factor, allows construction at the optimum cost. However, the difficulty of carrying out a complete probabilistic ana lysis has to be taken into account. For such an analysis t he laws of the distribution of the live load and its induc ed stresses, of the scatter of mechanical properties of mate rials, and of the geometry of the cross-sections and the st ructure have to be known. Furthermore, it is difficult to i nterpret the interaction between the law of distribution of strength and that of stresses because both depend upon the nature of the material, on the cross-sections and upon the load acting on the structure. These practical difficulties ca n be overcome in two ways. The first is to apply different safety factors to the material and to the loads, without necessarily adopting the probabilistic criterion. The second i s an approximate probabilistic method which introduces some s implifying assumptions ( semi-probabilistic methods ) . Aspart of mitigation programs to reduce the likelihood of mass casualties following local damage in structures, the General Services Administration [1] and the Department of Defense [2] developed regulations to evaluate progressive collapse resistance of structures. ASCE/SEI 7 [3] defines progressive collapse as the spread of an initial local failure fromelement to element eventually resulting in collapse of an entire structure or a disproportionately large part of it. Following the approaches proposed by Ellinwood and Leyendecker [4], ASCE/SEI 7 [3] defines two general methods for structural design of buildings to mitigate damage due to progressive collapse: indirect and direct design methods. General building codes and standards [3,5] use indirect design by increasing overall integrity of structures. Indirect design is also used in DOD [2]. Although the indirect design method can reduce the risk of progressive collapse [6,7] estimation of post-failure performance of structures designed based on such a method is not readily possible. One approach based on direct design methods to evaluate progressive collapse of structures is to study the effects of instantaneous removal of load-bearing elements, such as columns. GSA [1] and DOD [2] regulations require removal of one load bearing element. These regulations are meant to evaluate general integrity of structures and their capacity of redistributing the loads following severe damage to only one element. While such an approach provides insight as to the extent to which the structures are susceptible to progressive collapse, in reality, the initial damage can affect more than just one column. In this study, using analytical results that are verified against experimental data, the progressive collapse resistance of the Hotel San Diego is evaluated, following the simultaneous explosion (sudden removal) of two adjacent columns, one of which was a corner column. In order to explode the columns, explosives were inserted into predrilled holes in the columns. The columns were then well wrapped with a few layers of protective materials. Therefore, neither air blast nor flying fragments affected the structure.2. Building characteristicsHotel San Diego was constructed in 1914 with a south annex added in 1924. The annex included two separate buildings. Fig. 1 shows a south view of the hotel. Note that in the picture, the first and third stories of the hotel are covered with black fabric. The six story hotel had a non-ductile reinforced concrete (RC) frame structure with hollow clay tile exterior infill walls. The infills in the annex consisted of two withes (layers) of clay tiles with a total thickness of about 8 in (203 mm). The height of the first floor was about 190–800 m). The height of other floors and that of the top floor were 100–600 m) and 160–1000 m), respectively. Fig. 2 shows the second floor of one of the annex buildings. Fig. 3 shows a typical plan of this building, whose responsefollowing the simultaneous removal (explosion) of columns A2 and A3 in the first (ground) floor is evaluated in this paper. The floor system consisted of one-way joists running in the longitudinal direction (North–South), as shown in Fig. 3. Based on compression tests of two concrete samples, the average concrete compressive strength was estimated at about 4500 psi (31 MPa) for a standard concrete cylinder. The modulus of elasticity of concrete was estimated at 3820 ksi (26 300 MPa) [5]. Also, based on tension tests of two steel samples having 1/2 in mm) square sections, the yield and ultimate tensile strengths were found to be 62 ksi (427 MPa) and 87 ksi (600 MPa), respectively. The steel ultimate tensile strain was measured at . The modulus of elasticity of steel was set equal to 29 000 ksi (200 000 MPa). The building was scheduled to be demolished by implosion. As part of the demolition process, the infill walls were removed from the first and third floors. There was no live load in the building. All nonstructural elements including partitions, plumbing, and furniture were removed prior to implosion. Only beams, columns, joist floor and infill walls on the peripheral beams were present.3. SensorsConcrete and steel strain gages were used to measure changes in strains of beams and columns. Linear potentiometers were used to measure global and local deformations. The concrete strain gages were in (90 mm) long having a maximum strain limit of ±. The steel strain gages could measure up to a strain of ±. The strain gages could operate up to a several hundred kHz sampling rate. The sampling rate used in the experiment was 1000 Hz. Potentiometers were used to capture rotation (integral of curvature over a length) of the beam end regions and global displacementin the building, as described later. The potentiometers had a resolution of about in mm) and a maximum operational speed of about 40 in/s m/s), while the maximum recorded speed in the experiment was about 14 in/sm/s).4. Finite element modelUsing the finite element method (FEM), a model of the building was developed in the SAP2000 [8] computer program. The beams and columns are modeled with Bernoulli beam elements. Beams have T or L sections with effective flange width on each side of the web equal to four times the slab thickness [5]. Plastic hinges are assigned to all possible locations where steel bar yielding can occur, including the ends of elements as well as the reinforcing bar cut-off and bend locations. The characteristics of the plastic hinges are obtained using section analysesof the beams and columns and assuming a plastic hinge length equal to half of the section depth. The current version of SAP2000 [8] is not able to track formation of cracks in the elements. In order to find the proper flexural stiffness of sections, an iterative procedure is used as follows. First, the building is analyzed assuming all elements are uncracked. Then, moment demands in the elements are compared with their cracking bending moments, Mcr . The moment of inertia of beam and slab segments are reduced by a coefficient of [5], where the demand exceeds the Mcr. The exterior beam cracking bending moments under negative and positive moments, are 516 k in kN m) and 336 k in kN m), respectively. Note that no cracks were formed in the columns. Then the building is reanalyzed and moment diagrams are re-evaluated. This procedure is repeated until all of the cracked regions are properly identified and modeled.The beams in the building did not have top reinforcing bars except at the end regions (see Fig. 4). For instance, no top reinforcement was provided beyond the bend in beam A1–A2, 12 inches away from the face of column A1 (see Figs. 4 and 5). To model the potential loss of flexural strength in those sections, localized crack hinges were assigned at the critical locations where no top rebar was present. Flexural strengths of the hinges were set equal to Mcr. Such sections were assumed to lose their flexural strength when the imposed bending moments reached Mcr.The floor system consisted of joists in the longitudinal direction (North–South). Fig. 6 shows the cross section of a typical floor. In order to account for potential nonlinear response of slabs and joists, floors are molded by beam elements. Joists are modeled with T-sections, having effective flange width on each side of the web equal to four times the slab thickness [5]. Given the large joist spacing between axes 2 and 3, two rectangular beam elements with 20-inch wide sections are used between the joist and the longitudinal beams of axes 2 and 3 to model the slab in the longitudinal direction. To model the behavior of the slab in the transverse direction, equally spaced parallel beams with 20-inch wide rectangular sections are used. There is a difference between the shear flow in the slab and that in the beam elements with rectangular sections modeling the slab. Because of this, the torsional stiffness is setequal to one-half of that of the gross sections [9].The building had infill walls on 2nd, 4th, 5th and 6th floors on the spandrel beams with some openings . windows and doors). As mentioned before and as part of the demolition procedure, the infill walls in the 1st and 3rd floors were removed before the test. The infill walls were made of hollow clay tiles, which were in good condition. The net area of the clay tiles was about 1/2 of the gross area. The in-plane action of the infill walls contributes to the building stiffness and strength and affects the building response. Ignoring the effects of the infill walls and excluding them in the model would result in underestimating the building stiffness and strength.Using the SAP2000 computer program [8], two types of modeling for the infills are considered in this study: one uses two dimensional shell elements (Model A) and the other uses compressive struts (Model B) as suggested in FEMA356 [10] guidelines.. Model A (infills modeled by shell elements)Infill walls are modeled with shell elements. However, the current version of the SAP2000 computer program includes only linear shell elements and cannot account for cracking. The tensile strength of the infill walls is set equal to 26 psi, with a modulus of elasticity of 644 ksi [10]. Because the formation ofcracks has a significant effect on the stiffness of the infill walls, the following iterative procedure is used to account for crack formation:(1) Assuming the infill walls are linear and uncracked, a nonlinear time history analysis is run. Note that plastic hinges exist in the beam elements and the segments of the beam elements where moment demand exceeds the cracking moment have a reduced moment of inertia.(2) The cracking pattern in the infill wall is determined by comparingstresses in the shells developed during the analysis with the tensile strength of infills.(3) Nodes are separated at the locations where tensile stress exceeds tensile strength. These steps are continued until the crack regions are properly modeled.. Model B (infills modeled by struts)Infill walls are replaced with compressive struts as described in FEMA 356 [10] guidelines. Orientations of the struts are determined from the deformed shape of the structure after column removal and the location of openings.. Column removalRemoval of the columns is simulated with the following procedure. (1) The structure is analyzed under the permanent loads and the internal forces are determined at the ends of the columns, which will be removed.(2) The model is modified by removing columns A2 and A3 on the first floor. Again the structure is statically analyzed under permanent loads. In this case, the internal forces at the ends of removed columns found in the first step are applied externally to the structure along with permanent loads. Note that the results of this analysis are identical to those of step 1.(3) The equal and opposite column end forces that were applied in the second step are dynamically imposed on the ends of the removed column within one millisecond [11] to simulate the removal of the columns, and dynamic analysis is conducted.. Comparison of analytical and experimental resultsThe maximum calculated vertical displacement of the building occurs at joint A3 in the second floor. Fig. 7 shows the experimental andanalytical (Model A) vertical displacements of this joint (the AEM results will be discussed in the next section). Experimental data is obtained using the recordings of three potentiometers attached to joint A3 on one of their ends, and to the ground on the other ends. The peak displacements obtained experimentally and analytically (Model A) are in mm) and in mm), respectively, which differ only by about 4%. The experimental and analytical times corresponding to peak displacement are s and s, respectively. The analytical results show a permanent displacement of about in mm), which is about 14% smaller than the corresponding experimental value of in mm).Fig. 8 compares vertical displacement histories of joint A3 in the second floor estimated analytically based on Models A and B. As can be seen, modeling infills with struts (Model B) results in a maximum vertical displacement of joint A3 equal to about in mm), which is approximately 80% larger than the value obtained from Model A. Note that the results obtained from Model A are in close agreement with experimental results (see Fig. 7), while Model B significantly overestimates the deformation of the structure. If the maximum vertical displacement were larger, the infill walls were more severely cracked and the struts were more completely formed, the difference between the results of the two models (Models A and B) would be smaller.Fig. 9 compares the experimental and analytical (Model A) displacement of joint A2 in the second floor. Again, while the first peak vertical displacement obtained experimentally and analytically are in good agreement, the analytical permanent displacement under estimates the experimental value.Analytically estimated deformed shapes of the structure at the maximumvertical displacement based on Model A are shown in Fig. 10 with a magnification factor of 200. The experimentally measured deformed shape over the end regions of beams A1–A2 and A3–B3 in the second floorare represented in the figure by solid lines. A total of 14 potentiometers were located at the top and bottom of the end regions of the second floor beams A1–A2 and A3–B3, which were the most critical elements in load redistribution. The beam top and corresponding bottom potentiometerrecordings were used to calculate rotation between the sections where the potentiometer ends were connected. This was done by first finding the difference between the recorded deformations at the top and bottom of the beam, and then dividing the value by the distance (along the height of the beam section) between the two potentiometers. The expected deformed shapes between the measured end regions of the second floor beams are shown by dashed lines. As can be seen in the figures, analytically estimated deformed shapes of the beams are in good agreement with experimentally obtained deformed shapes.Analytical results of Model A show that only two plastic hinges are formed indicating rebar yielding. Also, four sections that did not have negative (top) reinforcement, reached cracking moment capacities and therefore cracked. Fig. 10 shows the locations of all the formed plastic hinges and cracks.。
土木外文翻译原文和译文
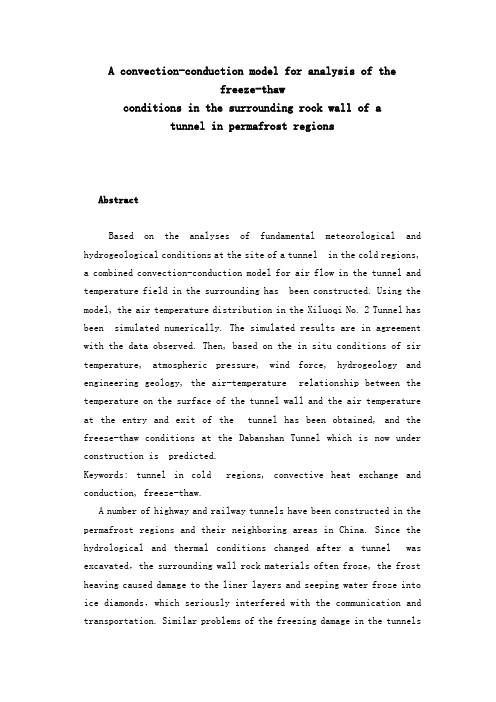
A convection-conduction model for analysis of thefreeze-thawconditions in the surrounding rock wall of atunnel in permafrost regionsAbstractBased on the analyses of fundamental meteorological and hydrogeological conditions at the site of a tunnel in the cold regions, a combined convection-conduction model for air flow in the tunnel and temperature field in the surrounding has been constructed. Using the model, the air temperature distribution in the Xiluoqi No. 2 Tunnel has been simulated numerically. The simulated results are in agreement with the data observed. Then, based on the in situ conditions of sir temperature, atmospheric pressure, wind force, hydrogeology and engineering geology, the air-temperature relationship between the temperature on the surface of the tunnel wall and the air temperature at the entry and exit of the tunnel has been obtained, and the freeze-thaw conditions at the Dabanshan Tunnel which is now under construction is predicted.Keywords: tunnel in cold regions, convective heat exchange and conduction, freeze-thaw.A number of highway and railway tunnels have been constructed in the permafrost regions and their neighboring areas in China. Since the hydrological and thermal conditions changed after a tunnel was excavated,the surrounding wall rock materials often froze, the frost heaving caused damage to the liner layers and seeping water froze into ice diamonds,which seriously interfered with the communication and transportation. Similar problems of the freezing damage in the tunnelsalso appeared in other countries like Russia, Norway and Japan .Hence it is urgent to predict the freeze-thaw conditions in the surrounding rock materials and provide a basis for the design,construction and maintenance of new tunnels in cold regions.Many tunnels,constructed in cold regions or their neighbouring areas,pass through the part beneath the permafrost base .After a tunnel is excavated,the original thermodynamical conditions in the surroundings are and thaw destroyed and replaced mainly by the air connections without the heat radiation, the conditions determined principally by the temperature and velocity of air flow in the tunnel,the coefficients of convective heat transfer on the tunnel wall,and the geothermal heat. In order to analyze and predict the freeze and thaw conditions of the surrounding wall rock of a tunnel,presuming the axial variations of air flow temperature and the coefficients of convective heat transfer, Lunardini discussed the freeze and thaw conditions by the approximate formulae obtained by Sham-sundar in study of freezing outside a circular tube with axial variations of coolant temperature .We simulated the temperature conditions on the surface of a tunnel wall varying similarly to the periodic changes of the outside air temperature .In fact,the temperatures of the air and the surrounding wall rock material affect each other so we cannot find the temperature variations of the air flow in advance; furthermore,it is difficult to quantify the coefficient of convective heat exchange at the surface of the tunnel wall .Therefore it is not practicable to define the temperature on the surface of the tunnel wall according to the outside air temperature .In this paper, we combine the air flow convective heat ex-change and heat conduction in the surrounding rock material into one model,and simulate the freeze-thaw conditions of the surrounding rock material based on the in situ conditions of air temperature,atmospheric pressure,wind force at the entry and exit of the tunnel,and the conditions of hydrogeology and engineering geology.重庆交通大学土木工程专业(隧道与城市轨道交通工程方向)毕业设计外文翻译Mathematical modelIn order to construct an appropriate model, we need the in situ fundamental conditions as a ba-sis .Here we use the conditions at the scene of the Dabanshan Tunnel. The Dabanshan Tunnel is lo-toted on the highway from Xining to Zhangye, south of the Datong River, at an elevation of 3754.78-3 801.23 m, with a length of 1 530 m and an alignment from southwest to northeast. The tunnel runs from the southwest to the northeast.Since the monthly-average air temperature is beneath 0`}C for eight months at the tunnel site each year and the construction would last for several years,the surrounding rock materials would become cooler during the construction .We conclude that, after excavation, the pattern of air flow would depend mainly on the dominant wind speed at the entry and exit,and the effects of the temperature difference between the inside and outside of the tunnel would be very small .Since the dominant wind direction is northeast at the tunnel site in winter, the air flow in the tunnel would go from the exit to the entry. Even though the dominant wind trend is southeastly in summer, considering the pressure difference, the temperature difference and the topography of the entry and exit,the air flow in the tunnel would also be from the exit to entry .Additionally,since the wind speed at the tunnel site is low,we could consider that the air flow would be principally laminar.Based on the reasons mentioned,we simplify the tunnel to a round tube,and consider that theair flow and temperature are symmetrical about the axis of the tunnel,Ignoring the influence of the air temperature on the speed of air flow, we obtain the following equation:where t ,x ,r are the time ,axial and radial coordinates; U ,V are axial and radial wind speeds; T is temperature; p is the effective pressure(that is ,air pressure divided by air density); v is the kinematic viscosity of air; a is the thermal conductivity of air; L is the length of the tunnel; R is the equivalent radius of the tunnel section; D is the length of time after the tunnel construction;,f S (t), u S (t) are frozen and thawed parts in the surrounding rock materials respectively; f λ,u λand f C ,u C are thermal conductivities and volumetric thermal capacities in frozen and thawed parts respectively; X= (x , r),ξ(t) is phase change front; Lh is heat latent of freezing water; and To is critical freezing temperature of rock ( here we assume To= -0.1℃).2 used for solving the modelEquation(1)shows flow. We first solve those concerning temperature at that the temperature of the surrounding rock does not affect the speed of air equations concerning the speed of air flow, and then solve those equations every time elapse.2. 1 Procedure used for solving the continuity and momentum equations重庆交通大学土木工程专业(隧道与城市轨道交通工程方向)毕业设计外文翻译Since the first three equations in(1) are not independent we derive the second equation by xand the third equation by r. After preliminary calculation we obtain the following elliptic equation concerning the effective pressure p:Then we solve equations in(1) using the following procedures:(i ) Assume the values for U0,V0;( ii ) substituting U0,V0 into eq. (2),and solving (2),we obtain p0;(iii) solving the first and second equations of(1),we obtain U0,V1;(iv) solving the first and third equations of(1),we obtain U2,V2; (v) calculating the momentum-average of U1,v1 and U2,v2,we obtain the new U0,V0;then return to (ii);(vi) iterating as above until the disparity of those solutions in two consecutive iterations is sufficiently small or is satisfied,we then take those values of p0,U0 and V0 as the initial values for the next elapse and solve those equations concerning the temperature..2 .2 Entire method used for solving the energy equationsAs mentioned previously,the temperature field of the surrounding rock and the air flow affect each other. Thus the surface of the tunnel wall is both the boundary of the temperature field in the surrounding rock and the boundary of the temperature field in air flow .Therefore, it is difficult to separately identify the temperature on the tunnel wall surface,and we cannot independently solve those equations concerning the temperature of air flow and those equations concerning the temperature of the surrounding rock .In order to cope with this problem,we simultaneously solve the two groups of equations based on the fact that at the tunnel wall surface both temperatures are equal .We should bearin mind the phase change while solving those equations concerning the temperature of the surrounding rock ,and the convection while solving those equations concerning the temperature of the air flow, and we only need to smooth those relative parameters at the tunnel wall surface .The solving methods for the equations with the phase change are the same as in reference [3].2.3 Determination of thermal parameters and initial and boundaryconditions2.3.1 Determination of the thermal parameters. Using p= 1013.25-0.1088 H ,we calculateair pressure p at elevation H and calculate the air density ρ using formula GTP =ρ, where T is the yearly-average absolute air temperature ,and G is the humidity constant of air. Letting P C be the thermal capacity with fixed pressure, λ the thermal conductivity ,and μ the dynamic viscosity of air flow, we calculate the thermal conductivity and kinematic viscosity using the formulas ρλP C =a and ρμν=. The thermal parameters of the surrounding rock are determined from the tunnel site.2 .3.2 Determination of the initial and boundary conditions .Choose the observed monthly average wind speed at the entry and exit as boundary conditions of wind speed ,and choose the relative effective pressure p=0 at the exit ( that is ,the entry of the dominant wind trend) and ]5[22/)/1(v d kL p ⨯+= on the section of entry ( that is ,the exit of the dominant wind trend ),where k is the coefficient of resistance along the tunnel wall, d = 2R ,and v is the axial average speed. We approximate T varying by the sine law according to the data observed at the scene and provide a suitable boundary value based on the position of the permafrost base and the geothermal gradient of the thaw rock materials beneath the重庆交通大学土木工程专业(隧道与城市轨道交通工程方向)毕业设计外文翻译permafrost base.3 A simulated exampleUsing the model and the solving method mentioned above,we simulate the varying law of the air temperature in the tunnel along with the temperature at the entry and exit of the Xiluoqi No.2 Tunnel .We observe that the simulated results are close to the data observed[6].The Xiluoqi No .2 Tunnel is located on the Nongling railway in northeastern China and passes through the part beneath the permafrost base .It has a length of 1 160 m running from the northwest to the southeast, with the entry of the tunnel in the northwest,and the elevation is about 700 m. The dominant wind direction in the tunnel is from northwest to southeast, with a maximum monthly-average speed of 3 m/s and a minimum monthly-average speed of 1 .7 m/s . Based on the data observed,we approximate the varying sine law of air temperature at the entry and exit with yearly averages of -5℃,-6.4℃ and amplitudes of 18.9℃ and 17.6℃respectively. The equivalent diameter is 5 .8m,and the resistant coefficient along the tunnel wall is 0.025.Since the effect of the thermal parameter of the surrounding rock on the air flow is much smaller than that of wind speed,pressure and temperature at the entry and exit,we refer to the data observed in the Dabanshan Tunnel for the thermal parameters.Figure 1 shows the simulated yearly-average air temperature inside and at the entry and exit of the tunnel compared with the data observed .We observe that the difference is less than 0 .2 `C from the entry to exit.Figure 2 shows a comparison of the simulated and observed monthly-average air temperature in-side (distance greater than 100 m from the entry and exit) the tunnel. We observe that the principal law is almost the same,and the main reason for the difference is the errors that came from approximating the varying sine law at the entry and exit; especially , the maximum monthly-average air temperature of 1979 was not for July but for August.Fig.1. Comparison of simulated and observed air temperature in Xiluoqi No.2 Tunnel in 1979.1,simulated values;2,observed valuesFig.2.The comparison of simulated and observed air temperature inside The Xiluoqi No.2 Tunnel in 1979.1,simulated values;2,observed values4 Prediction of the freeze-thaw conditions for the Dabanshan Tunnel 4 .1 Thermal parameter and initial and boundary conditionsUsing the elevation of 3 800 m and the yearly-average air temperature of -3℃, we calculate the air density p=0 .774 kg/m 3.Since steam exists In the air, we choose the thermal capacity with a fixed pressure of air ),./(8744.10C kg kJ C p = heat conductivity )./(100.202C m W -⨯=λ andand the dynamic viscosity )../(10218.96s m kg -⨯=μ After calculation we obtain the thermal diffusivity a= 1 .3788s m /1025-⨯ and the kinematic viscosity ,s m /1019.125-⨯=ν .Considering that the section of automobiles is much smaller than that of the tunnel and the auto-mobiles pass through the tunnel at a low speed ,we ignore the piston effects ,coming from the movement of automobiles ,in the diffusion of the air.We consider the rock as a whole component and choose the dry volumetric cavity 3/2400m kg d =λ,content of water and unfrozen water W=3% and W=1%, and the thermal conductivity c m W o u ./9.1=λ,c m W o f ./0.2=λ,heat重庆交通大学土木工程专业(隧道与城市轨道交通工程方向)毕业设计外文翻译capacityc kg kJ C o V ./8.0= and d u f W w C γ⨯++=1)128.48.0(,d u u Ww C γ⨯++=1)128.48.0( According to the data observed at the tunnel site ,the maximum monthly-average wind speed is about 3 .5 m/s ,and the minimum monthly-average wind speed is about 2 .5 m/s .We approximate the wind speed at the entry and exit as )/](5.2)7(028.0[)(2s m t t v +-⨯=, where t is in month. The initial wind speed in the tunnel is set to be.0),,0(),)(1(),,0(2=-=r x V R r U r x U a The initial and boundary values of temperature T are set to bewhere f(x) is the distance from the vault to the permafrost base ,and R0=25 m is the radius of do-main of solution T. We assume that the geothermal gradient is 3%,the yearly-average air temperature outside tunnel the is A=-3C 0,and the amplitude is B=12C 0.As for the boundary of R=Ro ,we first solve the equations considering R=Ro as the first type of boundary; that is we assume that T=f(x)⨯3%C 0on R=Ro. We find that, after one year, the heat flow trend will have changed in the range of radius between 5 and 25m in the surrounding rock.. Considering that the rock will be cooler hereafter and it will be affected yet by geothermal heat, we appoximately assume that the boundary R=Ro is the second type of boundary; that is ,we assume that the gradient value ,obtained from the calculation up to the end of the first year after excavation under the first type of boundary value, is the gradient on R=Ro of T.Considering the surrounding rock to be cooler during the period of construction ,we calculatefrom January and iterate some elapses of time under the same boundary. Then we let the boundaryvalues vary and solve the equations step by step(it can be proved that the solution will not depend on the choice of initial values after many time elapses ).1)The yearly-average temperature on the surface wall of the tunnel is approximately equal to the ai4 .2 Calculated resultsFigures 3 and 4 show the variations of the monthly-average temperatures on the surface of the tunnel wall along with the variations at the entry and exit .Figs .5 and 6 show the year when permafrost begins to form and the maximum thawed depth after permafrost formed in different surrounding sections.Fig.3.The monthly-average temperature parison of the monthly- On the surface of Dabanshan Tunnel.I, average temperature on the surface The month,I=1,2,3,,,12 tunnel with that outside the tunnel. 1,inner temperature on the surface ;2,outside air temperatureFig.5.The year when permafrost Fig.6.The maximum thawed depth after Begins to from in different permafrost formed in different years Sections of the surroundingrock重庆交通大学土木工程专业(隧道与城市轨道交通工程方向)毕业设计外文翻译4 .3 Preliminary conclusionBased on the initial-boundary conditions and thermal parameters mentioned above, we obtain the following preliminary conclusions: r temperature at the entry and exit. It is warmer during the cold season and cooler during the warm season in the internal part (more than 100 m from the entry and exit) of the tunnel than at the entry and exit . Fig .1 shows that the internal monthly-average temperature on the surface of the tunnel wall is 1.2℃ higher in January, February and December, 1℃higher in March and October, and 1 .6℃ lower in June and August, and 2qC lower in July than the air temperature at the entry and exit. In other months the infernal temperature on the surface of the tunnel wall approximately equals the air temperature at the entry and exit.2) Since it is affected by the geothermal heat in the internal surrounding section,especially in the central part, the internal amplitude of the yearly-average temperature on the surface of the tunnel wall decreases and is 1 .6℃ lower than that at the entry and exit.3 ) Under the conditions that the surrounding rock is compact , without a great amount of under-ground water, and using a thermal insulating layer(as designed PU with depth of 0.05 m and heat conductivity λ=0.0216 W/m℃,FBT with depth of 0.085 m and heat conductivity λ=0.0517W/m℃),in the third year after tunnel construction,the surrounding rock will begin to form permafrost in the range of 200 m from the entry and exit .In the first and the second year after construction, the surrounding rock will begin to form permafrost in the range of 40 and 100m from the entry and exit respectively .In the central part,more than 200m from the entry and exit, permafrost will begin to form in the eighth year. Near the center of the tunnel,permafrost will appear in the 14-15th years. During the first and second years after permafrost formed,the maximum of annual thawed depth is large (especially in the central part of the surrounding rock section) and thereafter it decreases every year. The maximum of annual thawed depth will be stable until the 19-20th yearsand will remain in s range of 2-3 m.4) If permafrost forms entirely in the surrounding rock,the permafrost will provide a water-isolating layer and be favourable for communication and transportation .However, in the process of construction,we found a lot of underground water in some sections of the surrounding rock .It will permanently exist in those sections,seeping out water and resulting in freezing damage to the liner layer. Further work will be reported elsewhere.重庆交通大学土木工程专业(隧道与城市轨道交通工程方向)毕业设计外文翻译严寒地区隧道围岩冻融状况分析的导热与对流换热模型摘要通过对严寒地区隧道现场基本气象条件的分析,建立了隧道内空气与围岩对流换热及固体导热的综合模型;用此模型对大兴安岭西罗奇2号隧道的洞内气温分布进行了模拟计算,结果与实测值基本一致;分析预报了正在开凿的祁连山区大坂山隧道开通运营后洞内温度及围岩冻结、融化状况.关键词严寒地区隧道导热与对流换热冻结与融化在我国多年冻土分布及邻近地区,修筑了公路和铁路隧道几十座.由于隧道开通后洞内水热条件的变化;,普遍引起洞内围岩冻结,造成对衬砌层的冻胀破坏以及洞内渗水冻结成冰凌等,严重影响了正常交通.类似隧道冻害问题同样出现在其他国家(苏联、挪威、日本等)的寒冷地区.如何预测分析隧道开挖后围岩的冻结状况,为严寒地区隧道建设的设计、施工及维护提供依据,这是一个亟待解决的重要课题.在多年冻土及其临近地区修筑的隧道,多数除进出口部分外从多年冻土下限以下岩层穿过.隧道贯通后,围岩内原有的稳定热力学条件遭到破坏,代之以阻断热辐射、开放通风对流为特征的新的热力系统.隧道开通运营后,围岩的冻融特性将主要由流经洞内的气流的温度、速度、气—固交界面的换热以及地热梯度所确定.为分析预测隧道开通后围岩的冻融特性,Lu-nardini借用Shamsundar研究圆形制冷管周围土体冻融特性时所得的近似公式,讨论过围岩的冻融特性.我们也曾就壁面温度随气温周期性变化的情况,分析计算了隧道围岩的温度场[3].但实际情况下,围岩与气体的温度场相互作用,隧道内气体温度的变化规律无法预先知道,加之洞壁表面的换热系数在技术上很难测定,从而由气温的变化确定壁面温度的变化难以实现.本文通过气一固祸合的办法,把气体、固体的换热和导热作为整体来处理,从洞口气温、风速和空气湿度、压力及围岩的水热物理参数等基本数据出发,计算出围岩的温度场.1数学模型为确定合适的数学模型,须以现场的基本情况为依据.这里我们以青海祁连山区大坂山公路隧道的基本情况为背景来加以说明.大坂山隧道位于西宁一张业公路大河以南,海拔3754.78~3801.23 m ,全长1530 m ,隧道近西南—东北走向. 由于大坂山地区隧道施工现场平均气温为负温的时间每年约长8个月,加之施工时间持续数年,围岩在施土过程中己经预冷,所以隧道开通运营后,洞内气体流动的形态主要由进出口的主导风速所确定,而受洞内围岩地温与洞外气温的温度压差的影响较小;冬季祁连山区盛行西北风,气流将从隧道出曰流向进口端,夏季虽然祁连山区盛行东偏南风,但考虑到洞口两端气压差、温度压差以及进出口地形等因素,洞内气流仍将由出口北端流向进口端.另外,由于现场年平均风速不大,可以认为洞内气体将以层流为主基于以上基本情况,我们将隧道简化成圆筒,并认为气流、温度等关十隧道中心线轴对称,忽略气体温度的变化对其流速的影响,可有如下的方程:其中t 为时间,x 为轴向坐标,r 为径向坐标;U, V 分别为轴向和径向速度,T 为温度,P 为有效压力(即空气压力与空气密度之比少,V 为空气运动粘性系数,a 为空气的导温系数,L 为隧道长度,R 为隧道的当量半径,D 为时间长度)(t S f , )(t S u 分别为围岩的冻、融区域. f λ,u λ分别为冻、融状态下的热传导系数,f C ,u C 分别为冻、融状态下的体积热容量,X=(x,r) , )(t ξ为冻、融相变界面,To 为岩石冻结临界温度(这里具体计算时取To=-0.10C 0),h L 为水的相变潜热.重庆交通大学土木工程专业(隧道与城市轨道交通工程方向)毕业设计外文翻译2 求解过程由方程(1)知,围岩的温度的高低不影响气体的流动速度,所以我们可先解出速度,再解温度.2.1 连续性方程和动量方程的求解由于方程((1)的前3个方程不是相互独立的,通过将动量方程分别对x 和r 求导,经整理化简,我们得到关于压力P 的如下椭圆型方程:于是,对方程(1)中的连续性方程和动量方程的求解,我们按如下步骤进行:(1)设定速度0U ,0V ;( 2)将0U ,0V 代入方程并求解,得0P(3)联立方程(1)的第一个和第二个方程,解得一组解1U ,1V ;(4)联立方程((1)的第一个和第三个方程,解得一组解2U ,2V ;(5)对((3) ,(4)得到的速度进行动量平均,得新的0U ,0V 返回(2) ;(6)按上述方法进行迭代,直到前后两次的速度值之差足够小.以0P ,0U ,0V 作为本时段的解,下一时段求解时以此作为迭代初值.2. 2 能量方程的整体解法如前所述,围岩与空气的温度场相互作用,壁面既是气体温度场的边界,又是固体温度场的边界,壁面的温度值难以确定,我们无法分别独立地求解隧道内的气体温度场和围岩温度场.为克服这一困难,我们利用在洞壁表面上,固体温度等于气体温度这一事实,把隧道内气体的温度和围岩内固体的温度放在一起求解,这样壁面温度将作为末知量被解出来.只是需要注意两点:解流体温度场时不考虑相变和解固体温度时没有对流项;在洞壁表面上方程系数的光滑化.另外,带相变的温度场的算法与文献[3]相同.2. 3热参数及初边值的确定热参数的确定方法: 用p=1013.25-0.1088H 计算出海拔高度为H 的隧道现场的大气压强,再由GT P =ρ计算出现场空气密度ρ,其中T 为现场大气的年平均绝对温度,G 为空气的气体常数.记定压比热为P C ,导热系数为λ,空气的动力粘性系数为μ.按ρλP C =a 和ρμν= 计算空气的导温系数和运动粘性系数.围岩的热物理参数则由现场采样测定.初边值的确定方法:洞曰风速取为现场观测的各月平均风速.取卞导风进曰的相对有效气压为0,主导风出口的气压则取为]5[22/)/1(v d kL p ⨯+=,这里k 为隧道内的沿程阻力系数,L 为隧道长度,d 为隧道端面的当量直径,ν为进口端面轴向平均速度.进出口气温年变化规律由现场观测资料,用正弦曲线拟合,围岩内计算区域的边界按现场多年冻土下限和地热梯度确定出适当的温度值或温度梯度. 3 计算实例按以上所述的模型及计算方法,我们对大兴安岭西罗奇2号隧道内气温随洞曰外气温变化的规律进行了模拟计算验证,所得结果与实测值[6]相比较,基本规律一致.西罗奇2号隧道是位十东北嫩林线的一座非多年冻土单线铁路隧道,全长1160 m ,隧道近西北一东南向,高洞口位于西北向,冬季隧道主导风向为西北风.洞口海拔高度约为700 m ,月平均最高风速约为3m/s,最低风速约为1.7m/s.根据现场观测资料,我们将进出口气温拟合为年平均分别为-5C 0和-6.4C 0,年变化振幅分别为18.9C 0和17.6C 0的正弦曲线.隧道的当量直径为5.8 m,沿程阻力系数取为0.025.由于围岩的热物理参数对计算洞内气温的影响远比洞口的风速、压力及气温的影响小得多,我们这里参考使用了大坂山隧道的资料.图1给出了洞口及洞内年平均气温的计算值与观测值比较的情况,从进口到出口,两值之差都小于0.2C 0.图2给出了洞内 (距进出口l00m 以上)月平均气温的计算值与观测值比较的情况,可以看出温度变化的基本规律完全一致,造成两值之差的主要原因是洞口气温年变化规律之正弦曲线的拟合误差,特别是1979年隧道现场月平均最高气温不是在7月份,而是在8月份.重庆交通大学土木工程专业(隧道与城市轨道交通工程方向)毕业设计外文翻译图1. 比较1979年在西罗奇周家山2号隧道仿真试验与观察的空气温度.1、模拟值;2、观测值图2。
土木工程专业英语课文原文及对照翻译

Civil EngineeringCivil engineering, the oldest of the engineering specialties, is the planning, design, construction, and management of the built environment. This environment includes all structures built according to scientific principles, from irrigation and drainage systems to rocket-launching facilities.土木工程学作为最老的工程技术学科,是指规划,设计,施工及对建筑环境的管理。
此处的环境包括建筑符合科学规范的所有结构,从灌溉和排水系统到火箭发射设施。
Civil engineers build roads, bridges, tunnels, dams, harbors, power plants, water and sewage systems, hospitals, schools, mass transit, and other public facilities essential to modern society and large population concentrations. They also build privately owned facilities such as airports, railroads, pipelines, skyscrapers, and other large structures designed for industrial, commercial, or residential use. In addition, civil engineers plan, design, and build complete cities and towns, and more recently have been planning and designing space platforms to house self-contained communities.土木工程师建造道路,桥梁,管道,大坝,海港,发电厂,给排水系统,医院,学校,公共交通和其他现代社会和大量人口集中地区的基础公共设施。
土木工程外文翻译(中英互译版)
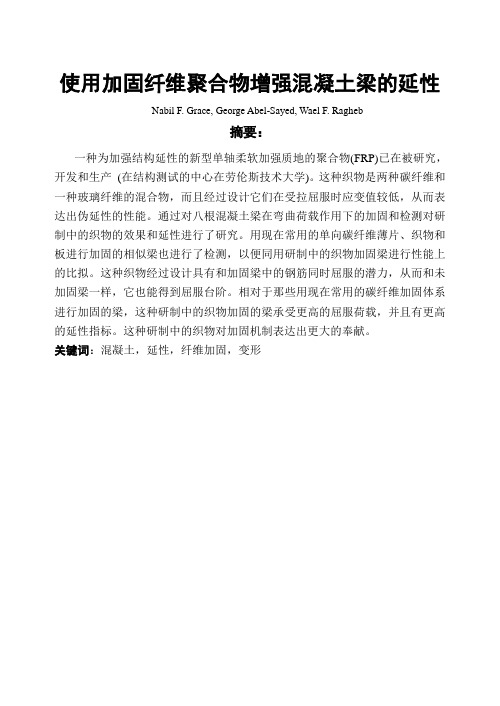
使用加固纤维聚合物增强混凝土梁的延性Nabil F. Grace, George Abel-Sayed, Wael F. Ragheb摘要:一种为加强结构延性的新型单轴柔软加强质地的聚合物(FRP)已在被研究,开发和生产(在结构测试的中心在劳伦斯技术大学)。
这种织物是两种碳纤维和一种玻璃纤维的混合物,而且经过设计它们在受拉屈服时应变值较低,从而表达出伪延性的性能。
通过对八根混凝土梁在弯曲荷载作用下的加固和检测对研制中的织物的效果和延性进行了研究。
用现在常用的单向碳纤维薄片、织物和板进行加固的相似梁也进行了检测,以便同用研制中的织物加固梁进行性能上的比拟。
这种织物经过设计具有和加固梁中的钢筋同时屈服的潜力,从而和未加固梁一样,它也能得到屈服台阶。
相对于那些用现在常用的碳纤维加固体系进行加固的梁,这种研制中的织物加固的梁承受更高的屈服荷载,并且有更高的延性指标。
这种研制中的织物对加固机制表达出更大的奉献。
关键词:混凝土,延性,纤维加固,变形介绍外贴粘合纤维增强聚合物〔FRP〕片和条带近来已经被确定是一种对钢筋混凝土结构进行修复和加固的有效手段。
关于应用外贴粘合FRP板、薄片和织物对混凝土梁进行变形加固的钢筋混凝土梁的性能,一些试验研究调查已经进行过报告。
Saadatmanesh和Ehsani〔1991〕检测了应用玻璃纤维增强聚合物(GFRP)板进行变形加固的钢筋混凝土梁的性能。
Ritchie等人〔1991〕检测了应用GFRP,碳纤维增强聚合物〔CFRP〕和G/CFRP板进行变形加固的钢筋混凝土梁的性能。
Grace等人〔1999〕和Triantafillou〔1992〕研究了应用CFRP薄片进行变形加固的钢筋混凝土梁的性能。
Norris,Saadatmanesh和Ehsani〔1997〕研究了应用单向CFRP薄片和CFRP织物进行加固的混凝土梁的性能。
在所有的这些研究中,加固的梁比未加固的梁承受更高的极限荷载。
土木工程专业英语课文原文及对照翻译

土木工程专业英语课文原文及对照翻译土木工程师建造道路、桥梁、隧道、水坝、港口、发电厂、水和污水系统、医院、学校、大众交通和其他对现代社会和大量人口集中地区至关重要的公共设施。
他们还建造私人拥有的设施,如机场、铁路、管道、摩天大楼和其他为工业、商业或住宅使用而设计的大型结构。
此外,土木工程师规划、设计和建造完整的城市和城镇,最近还在规划和设计太空平台,以容纳自给自足的社区。
___ passes the planning。
design。
n。
and management of the built ___ scientific principles。
from ___ are essential to modern society。
such as roads。
bridges。
___。
dams。
and hospitals.___ public facilities。
civil engineers also design and build privately-owned structures。
including airports。
railroads。
pipelines。
skyscrapers。
and other ___。
and ___.Overall。
___ civil engineers。
our modern infrastructure and public facilities would not exist.___。
n。
and maintenance of public and private infrastructure。
This includes roads。
bridges。
pipelines。
dams。
ports。
power plants。
water supply and sewage systems。
hospitals。
schools。
___。
and other structures that are essential to modern ___ as airports。
土木工程外文翻译5
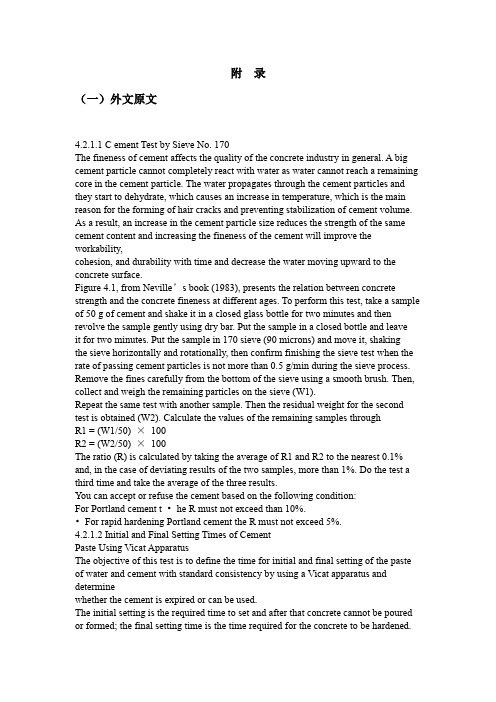
附录(一)外文原文4.2.1.1 C ement Test by Sieve No. 170The fineness of cement affects the quality of the concrete industry in general. A big cement particle cannot completely react with water as water cannot reach a remaining core in the cement particle. The water propagates through the cement particles and they start to dehydrate, which causes an increase in temperature, which is the main reason for the forming of hair cracks and preventing stabilization of cement volume. As a result, an increase in the cement particle size reduces the strength of the same cement content and increasing the fineness of the cement will improve the workability,cohesion, and durability with time and decrease the water moving upward to the concrete surface.Figure 4.1, from Neville’s book (1983), presents the relation between concrete strength and the concrete fineness at different ages. To perform this test, take a sample of 50 g of cement and shake it in a closed glass bottle for two minutes and then revolve the sample gently using dry bar. Put the sample in a closed bottle and leaveit for two minutes. Put the sample in 170 sieve (90 microns) and move it, shakingthe sieve horizontally and rotationally, then confirm finishing the sieve test when the rate of passing cement particles is not more than 0.5 g/min during the sieve process. Remove the fines carefully from the bottom of the sieve using a smooth brush. Then, collect and weigh the remaining particles on the sieve (W1).Repeat the same test with another sample. Then the residual weight for the second test is obtained (W2). Calculate the values of the remaining samples throughR1 = (W1/50) ×100R2 = (W2/50) ×100The ratio (R) is calculated by taking the average of R1 and R2 to the nearest 0.1% and, in the case of deviating results of the two samples, more than 1%. Do the test a third time and take the average of the three results.You can accept or refuse the cement based on the following condition:For Portland cement t •he R must not exceed than 10%.•For rapid hardening Portland cement the R must not exceed 5%.4.2.1.2 Initial and Final Setting Times of CementPaste Using Vicat ApparatusThe objective of this test is to define the time for initial and final setting of the paste of water and cement with standard consistency by using a Vicat apparatus and determinewhether the cement is expired or can be used.The initial setting is the required time to set and after that concrete cannot be poured or formed; the final setting time is the time required for the concrete to be hardened.Vicat apparatus (Figure 4.2) consists of a carrier with needle acting under a prescribed weight. The parts move vertically without friction and are not subject to erosionor corrosion. The paste mold is made from a metal or hard rubber or plastic likea cut cone with depth of 40 ±2 mm and the internal diameter of the upper face 70 ±5 mm and lower face 80 ±5 mm and provides a template of glass or similar materialsin the softer surface. Its dimensions are greater than the dimensions of the mold.The needle is used to determine the initial setting time in a steel cylinder with effective length 50 ±1 mm and diameter 1.13 ±0.5 mm. The needle measuring timeis in the form of a cylinder with length of 30 ±1 mm and diameter 1.13 ±0.5 mm andheld by a 5 mm diameter ring at the free end to achieve distance between the end of the needle and the ring of 0.5 mm.The test starts by taking a sample weighing about 400 g and placing it on an impermeable surface and then adding 100 ml of water and recording zero measurementfrom the time of adding water to the cement and then mixing for 240 + 5 secondson the impermeable surface.To determine the initial setting time and calibrate the device until the needle reaches the base of the mold, then adjust the measuring device to zero and return needle to its original place.Fill the mold with cement paste with standard consistency and troll the surface,then put the mold for a short time in a place that has the the temperature and humidity required for the test.Specific Surface (Wagner)-m2/kg365 days90 days28 days7 days20150 200 250 300304050Compressive Strength, MpaTransfer the mold to the apparatus under the needle, and then make the needleslowly approach the surface until it touches the paste’s surface, stop it in place fora second or two seconds to avoid impact of primary speed, then allow the moving parts to implement the needle vertically in the paste.Grading depends on when the needle stops penetrating or after 30 seconds, whichever is earlier, and indicates the distance between the mold base and the end of the needle, as well as the time start from the zero level measurement.Repeat the process of immersing the needle in the same paste in different locations with the distance between the immersing point and the edge of the mold orbetween two immersing points not less 10 mm after about 10 minutes, and clean the needle immediately after each test.Record time is measured from zero up to 5 ±1 mm from the base of the mold as the initial setting time to the nearest 5 minutes. Ensuring the accuracy of measurement of time between tests reduces embedment and the fluctuation of successivetests. The needle is used to identify the final time of setting; follow the same stepsas in determining time of initial setting and increase the period between embedment tests to 30 minutes.Record the time from zero measurement until embedment of the needle to a distance of 0.5 mm, which will be the final setting time. Control the impact of theneedle on the surface of the sample so the final setting time presents the effect ofthe needle. To enhance the test’s accuracy reduce the time between embedment tests and examine the fluctuation of these successive tests. Record the final setting timeto the nearest 5 mm.According to the Egyptian specifications the initial setting time must not be lessthan 45 minutes for all types of cement except the low heat cement, for which the initial setting time must not be less 60 minutes. The final setting time must be shorter than 10 hours for all types of cement.4.2.1.3 D ensity of CementThe purpose of this test is to determine the density of cement by identifying the weight and unit volume of the material by using the Le Chatelier density bottle. The determination of the cement density is essential for concrete mix design and to control its quality. This test follows specifications of the American Society for Testingand Materials, ASTM C188-84.The Le Chatelier device is a standard round bottle. Its shape and dimensions are shown in Figure 4.3. This bottle must have all the required dimensions, lengths, and uniform degradation and accuracy.The glass that is used in the Le Chatelier bottle must be of high quality and freefrom any defects. It should not interact with chemicals and have high resistance to heat and appropriate thickness to have a high resistance to crushing. Measurements start at the bottle’s neck and go from zero to 1 mL and from 18 to 24 mL with accuracyto 0.1 mL. Each bottle must have a number to distinguish it from any other.Write on the bottle the standard temperature and the capacity in millimeters over the highest point of grading.Processed sample cement weighing about 64 g to the nearest 0.05 g must be tested.Fill the bottle with kerosene free from water and oil whose density is at least 62 API. Up to point gradations between zero and 1 mL, dry the inner surface of the bottle at the highest level of kerosene if necessary, and use rubber on the surface of the table used for the test when filling the bottle.The bottle, which is filled with kerosene, is placed in a water bath and the firstreading to kerosene level is recorded. To record the first reading correctly install the bottle in the water bath vertically. Put a cement sample weighing 64 g with accuracy to 0.05 g inside the bottle with small batches at the same temperature of kerosene, taking into account when putting the cement inside the bottle to avoid cement droppingout or its adhesion on the internal surfaces of the bottle at the highest level. The bottle can be placed on the vibrating machine when putting the cement inside the bottle to expedite the process and prevent adhesion of granulated cement with the internal surfaces of the bottle.After laying the cement inside the bottle, put a cap on the bottle mouth and then spin diagonally on the surface so as to expel the air between the granules of cement, and continue moving the bottle until the emergence of air bubbles stops from the kerosene surface inside the bottle.Put the bottle in the water bath and then take the final reading, and record the reading at the lower surface of kerosene so as to avoid the impact of surface tension. For the first and final readings, make sure that the bottle is placed in a water bath with constant temperature for a period not to exceed the difference in temperature between the first and final readings of about 0.2°C.The difference between the first and final reading is the volume of the moving liquid by the cement sample.The volume of the moving liquid = final reading –first reading4.2.1.4 D efine Cement Fineness by Using Blaine ApparatusThis test is used to determine the surface area by comparing the test sample with the specific reference. The greater surface area increases the speed of concrete hardening and obtains early strength. This test determines the acceptance of the cement. There are many tests to define cement fineness and one is a Blaine apparatus as stated in many codes such as the Egyptian code.This test depends on calculating the surface area by comparing the sample test and the reference sample using a Blaine apparatus to determine the time required to pass a definite quantity of air inside a cement layer with defined dimensions and porosity.A Blaine apparatus is shown in Figure 4.4. The first step in testing is to determine the volume of the cement layer using mercury in the ring device of the Blaine apparatus.Cement is then added and by knowing the weight of the cement before andafter adding it as well as the mercury density, the volume of the cement layer can be calculated.V = W1 –W2/DmwhereV is the volume of cement layer, cm3.Fi gure 4.4 Blaine apparatus.Concrete Materials and Tests 111W1 is the weight of mercury in grams that fills the device to nearest (0.0 g).W2 is the weight of mercury in grams that fills the device to nearest (0.0 g).Dm is the density of the mercury (g/cm3). From tables, define the mercury densityat the average temperature of the test by using the manometer in the Blaine apparatus.From the previous equation:Sr is the reference cement surface area, (cm2/g).Dr is the reference cement density (g/cm3).Pr is the porosity of the cement layer.Ir is the air visciosity in the average temperature for reference cement test.Tr is the average time required for the manometer liquid to settle in two marksto nearest 0.2 sec.K is the Blaine apparatus constant factor defined by the previous equation by knowing the time needed to pass the air in the sample.To retest the sample, we calculate its surface area by using the following equation:Sc = Sr(Dr/Dc) *(Tc/Tr)^0.5According to the Egyptian code, the acceptance and refusal of cement is based on limites shown in Table 4.2.Table 4.2Cement Fineness Acceptance andRefusal LimitsCement TypesCement Fineness Not LessThan cm2/gmOrdinary Portland 2750Rapid hardening Portland 3500Sulfate resistant Portland 2800Low heat Portland 2800White Portland 2700Mixing sand Portland 30004100 fineness 4100Slag Portland 25004.2.1.5 C ompressive Strength of Cement MortarsThe cement mortar compressive strength test is performed using standard cubesof cement mortar mixed manually and compacted mechanically using a standard vibrating machine. This test is considered a refusal or acceptance determination. Compressive strength is one of the most important properties of concrete. The concrete gains its compressive strength from cement paste as a result of the interactionbetween the cement and water added to the mix. So it is critical to make sure that the cement used is the appropriate compressive strength. This test should be done to all types of cement.Needed for the test are stainless steel sieves with standard square holes opened 850 or 650 microns. Stainless steel does not react with cement and weighs 210 g. The vibrating machine has a weight of about 29 kg and the speed of vibration is about 12,000 vertical vibrations + 400 RPM and the moment of vibrating column is0.016 N.m.The mold of the test is a cube 70.7 ±1 mm, the surface area for each surface is500 mm2, the acceptable tolerance in leveling is about 0.03 mm, and the tolerance between paralleling for each face is about 0.06 mm.The mold is manufactured from materials that will not react with the cement mortar, and the base of the mold is made from steel that can prevent leaks of the mortaror water from the mold. The base is matched with the vibrating machine.The sand should contain a percentage of silica not less than 90% by weight andmust be washed and dried very well. Moreover, the humidity of the sand must not be more than 0.1% by weight for it to pass through a sieve with openings of 850 microns, and for it to pass through the standard sieve size of 600 microns it should not have more than 10% humidity by weight (Tables 4.3 and 4.4).After performing the tests, the standard cubes will be crushed within one day,which is about 24 ±0.5 hours, and three days in the limits of 72 ±1 hour, and afterseven days within 168 ±1 hour, and after 28 days within 672 ±1 hour.Table 4.5 illustrates the limits of acceptance and rejection according to the cement mortar compressive strength. Note from the table that there is more than one typeof high-alumina cement as the types vary according to the percentage of oxide alumina.The compressive strength after 28 days will not be considered accepted orrejected unless clearly stated in the contract between the supplier and the client(二)外文原文翻译4.2.1.1水泥试验筛170号水泥的优质一般影响混凝土行业的质量。
土木工程外文翻译-原文

外文原文Response of a reinforced concrete infilled-frame structure to removal of twoadjacent columnsMehrdad Sasani_Northeastern University, 400 Snell Engineering Center, Boston, MA 02115, UnitedStatesReceived 27 June 2007; received in revised form 26 December 2007; accepted 24January 2008Available online 19 March 2008AbstractThe response of Hotel San Diego, a six-story reinforced concrete infilled-frame structure, is evaluated following the simultaneous removal of two adjacent exterior columns. Analytical models of the structure using the Finite Element Method as well as the Applied Element Method are used to calculate global and local deformations. The analytical results show good agreement with experimental data. The structure resisted progressive collapse with a measured maximum vertical displacement of only one quarter of an inch (6.4 mm). Deformation propagation over the height of the structure and the dynamic load redistribution following the column removal are experimentally and analytically evaluated and described. The difference between axial and flexural wave propagations is discussed. Three-dimensional Vierendeel (frame) action of the transverse and longitudinal frames with the participation of infill walls is identified as the major mechanism for redistribution of loads in the structure. The effects of two potential brittle modes of failure (fracture of beam sections without tensile reinforcement and reinforcing bar pull out) are described. The response of the structure due to additional gravity loads and in the absence of infill walls is analytically evaluated.c 2008 Elsevier Ltd. All rights reserved.Keywords: Progressive collapse; Load redistribution; Load resistance; Dynamic response; Nonlinear analysis; Brittle failure1.IntroductionThe principal scope of specifications is to provide general principles and computation al methods in order to verify safety of structures. The “ safety factor ”, which accor ding to modern trends is independent of the nature and combination of the materials u sed, can usually be defined as the ratio between the conditions. This ratio is also prop ortional to the inverse of the probability ( risk ) of failure of the structure.Failure has to be considered not only as overall collapse of the structure but also as un serviceability or, according to a more precise. Common definition. As the reaching of a “ limit state ” which causes the construction not to accomplish the task it was desi gned for. There are two categories of limit state :(1)Ultimate limit sate, which corresponds to the highest value of the load-bearing cap acity. Examples include local buckling or global instability of the structure; failure of some sections and subsequent transformation of the structure into a mechanism; failure by fatigue; elastic or plastic deformation or creep that cause a substantial change of t he geometry of the structure; and sensitivity of the structure to alternating loads, to fire and to explosions.(2)Service limit states, which are functions of the use and durability of the structure. E xamples include excessive deformations and displacements without instability; early o r excessive cracks; large vibrations; and corrosion.Computational methods used to verify structures with respect to the different safety co nditions can be separated into:(1)Deterministic methods, in which the main parameters are considered as nonrandom parameters.(2)Probabilistic methods, in which the main parameters are considered as random para meters.Alternatively, with respect to the different use of factors of safety, computational meth ods can be separated into:(1)Allowable stress method, in which the stresses computed under maximum loads are compared with the strength of the material reduced by given safety factors.(2)Limit states method, in which the structure may be proportioned on the basis of its maximum strength. This strength, as determined by rational analysis, shall not be less than that required to support a factored load equal to the sum of the factored live load and dead load ( ultimate state ).The stresses corresponding to working ( service ) conditions with unfactored live and dead loads are compared with prescribed values ( service limit state ) . From the four possible combinations of the first two and second two methods, we can obtain some u seful computational methods. Generally, two combinations prevail:(1)deterministic methods, which make use of allowable stresses. (2)Probabilistic meth ods, which make use of limit states.The main advantage of probabilistic approaches is that, at least in theory, it is possible to scientifically take into account all random factors of safety, which are then combin ed to define the safety factor. probabilistic approaches depend upon :(1) Random distribution of strength of materials with respect to the conditions of fabri cation and erection ( scatter of the values of mechanical properties through out the str ucture ); (2) Uncertainty of the geometry of the cross-section sand of the structure ( fa ults and imperfections due to fabrication and erection of the structure );(3) Uncertainty of the predicted live loads and dead loads acting on the structure; (4)U ncertainty related to the approximation of the computational method used ( deviation of the actual stresses from computed stresses ). Furthermore, probabilistic theories me an that the allowable risk can be based on several factors, such as :(1) Importance of the construction and gravity of the damage by its failure; (2)Numbe r of human lives which can be threatened by this failure; (3)Possibility and/or likeliho od of repairing the structure; (4) Predicted life of the structure. All these factors are rel ated to economic and social considerations such as:(1) Initial cost of the construction;(2) Amortization funds for the duration of the construction;(3) Cost of physical and material damage due to the failure of the construction;(4) Adverse impact on society;(5) Moral and psychological views.The definition of all these parameters, for a given safety factor, allows constructio n at the optimum cost. However, the difficulty of carrying out a complete probabilistic analysis has to be taken into account. For such an analysis the laws of the distribution of the live load and its induced stresses, of the scatter of mechanical properties of mat erials, and of the geometry of the cross-sections and the structure have to be known. F urthermore, it is difficult to interpret the interaction between the law of distribution of strength and that of stresses because both depend upon the nature of the material, on t he cross-sections and upon the load acting on the structure. These practical difficulties can be overcome in two ways. The first is to apply different safety factors to the mate rial and to the loads, without necessarily adopting the probabilistic criterion. The seco nd is an approximate probabilistic method which introduces some simplifying assump tions ( semi-probabilistic methods ) . As part of mitigation programs to reduce the likelihood of mass casualties following local damage in structures, the General Services Administration [1] and the Department of Defense [2] developed regulations to evaluate progressive collapse resistance of structures. ASCE/SEI 7 [3] defines progressive collapse as the spread of an initial local failure from element to element eventually resulting in collapse of an entire structure or a disproportionately large part of it. Following the approaches proposed by Ellinwood and Leyendecker [4], ASCE/SEI 7 [3] defines two general methods for structural design of buildings to mitigate damage due to progressive collapse: indirect and direct design methods. General building codes and standards [3,5] use indirect design by increasing overall integrity of structures. Indirect design is also used in DOD [2]. Although the indirect design method can reduce the risk of progressive collapse [6,7] estimation of post-failure performance of structures designed based on such a method is not readily possible. One approach based on direct design methods to evaluate progressive collapse of structures is to study the effects of instantaneous removal of load-bearing elements, such as columns. GSA [1] and DOD [2] regulations require removal of one load bearing element. These regulations are meant to evaluate general integrity of structures and their capacity of redistributing the loads following severe damage to only one element. While such an approach provides insight as to the extent to which the structures are susceptible to progressive collapse, in reality, the initial damage can affect more than just one column. In this study, using analytical results that are verified against experimental data, the progressive collapse resistance of the Hotel San Diego is evaluated, following the simultaneous explosion (sudden removal) of two adjacent columns, one of which was a corner column. In order to explode the columns, explosives were inserted into predrilled holes in the columns. The columns were then well wrapped with a few layers of protective materials. Therefore, neither air blast nor flying fragments affected the structure.2. Building characteristicsHotel San Diego was constructed in 1914 with a south annex added in 1924. The annex included two separate buildings. Fig. 1 shows a south view of the hotel. Note that in the picture, the first and third stories of the hotel are covered with black fabric. The six story hotel had a non-ductile reinforced concrete (RC) frame structure with hollow clay tile exterior infill walls. The infills in the annex consisted of two withes (layers) of clay tiles with a total thickness of about 8 in (203 mm). The height of the first floor was about 190–800 (6.00 m). The height of other floors and that of the top floor were 100–600 (3.20 m) and 160–1000 (5.13 m), respectively. Fig. 2 shows the second floor of one of the annex buildings. Fig. 3 shows a typical plan of this building, whose response following the simultaneous removal (explosion) of columns A2 and A3 in the first (ground) floor is evaluated in this paper. The floor system consisted of one-way joists running in the longitudinal direction (North–South), as shown in Fig. 3. Based on compression tests of two concrete samples, the average concrete compressive strength was estimated at about 4500 psi (31 MPa) for a standard concrete cylinder. The modulus of elasticity of concrete was estimated at 3820 ksi (26 300 MPa) [5]. Also, based on tension tests of two steel samples having 1/2 in (12.7 mm) square sections, the yield and ultimate tensile strengths were found to be 62 ksi (427 MPa) and 87 ksi (600 MPa), respectively. The steel ultimate tensile strain was measured at 0.17. The modulus of elasticity of steel was set equal to 29 000 ksi (200000 MPa). The building was scheduled to be demolished by implosion. As part of the demolition process, the infill walls were removed from the first and third floors. There was no live load in the building. All nonstructural elements including partitions, plumbing, and furniture were removed prior to implosion. Only beams, columns, joist floor and infill walls on the peripheralbeams were present.3. SensorsConcrete and steel strain gages were used to measure changes in strains of beams and columns. Linear potentiometers were used to measure global and local deformations. The concrete strain gages were 3.5 in (90 mm) long having a maximum strain limit of ±0.02. The steel strain gages could measure up to a strain of ±0.20. The strain gages could operate up to a several hundred kHz sampling rate. The sampling rate used in the experiment was 1000 Hz. Potentiometers were used to capture rotation (integral of curvature over a length) of the beam end regions and global displacement in the building, as described later. The potentiometers had a resolution of about 0.0004 in (0.01 mm) and a maximum operational speed of about 40 in/s (1.0 m/s), while the maximum recorded speed in the experiment was about 14 in/s (0.35m/s).4. Finite element modelUsing the finite element method (FEM), a model of the building was developed in the SAP2000 [8] computer program. The beams and columns are modeled with Bernoulli beam elements. Beams have T or L sections with effective flange width on each side of the web equal to four times the slab thickness [5]. Plastic hinges are assigned to all possible locations where steel bar yielding can occur, including the ends of elements as well as the reinforcing bar cut-off and bend locations. The characteristics of the plastic hinges are obtained using section analyses of the beams and columns and assuming a plastic hinge length equal to half of the section depth. The current version of SAP2000 [8] is not able to track formation of cracks in the elements. In order to find the proper flexural stiffness of sections, an iterative procedure is used as follows. First, the building is analyzed assuming all elements are uncracked. Then, moment demands in the elements are compared with their cracking bending moments, Mcr . The moment of inertia of beam and slab segments are reduced by a coefficient of 0.35 [5], where the demand exceeds the Mcr. The exteriorbeam cracking bending moments under negative and positive moments, are 516 k in (58.2 kN m) and 336 k in (37.9 kN m), respectively. Note that no cracks were formed in the columns. Then the building is reanalyzed and moment diagrams are re-evaluated. This procedure is repeated until all of the cracked regions are properly identified and modeled.The beams in the building did not have top reinforcing bars except at the end regions (see Fig. 4). For instance, no top reinforcement was provided beyond the bend in beam A1–A2, 12 inches away from the face of column A1 (see Figs. 4 and 5). To model the potential loss of flexural strength in those sections, localized crack hinges were assigned at the critical locations where no top rebar was present. Flexural strengths of the hinges were set equal to Mcr. Such sections were assumed to lose their flexural strength when the imposed bending moments reached Mcr.The floor system consisted of joists in the longitudinal direction (North–South). Fig. 6 shows the cross section of a typical floor. In order to account for potential nonlinear response of slabs and joists, floors are molded by beam elements. Joists are modeled with T-sections, having effective flange width on each side of the web equal to four times the slab thickness [5]. Given the large joist spacing between axes 2 and 3, two rectangular beam elements with 20-inch wide sections are used between the joist and the longitudinal beams of axes 2 and 3 to model the slab in the longitudinal direction. To model the behavior of the slab in the transverse direction, equally spaced parallel beams with 20-inch wide rectangular sections are used. There is a difference between the shear flow in the slab and that in the beam elements with rectangular sections modeling the slab. Because of this, the torsional stiffness is setequal to one-half of that of the gross sections [9].The building had infill walls on 2nd, 4th, 5th and 6th floors on the spandrel beamswith some openings (i.e. windows and doors). As mentioned before and as part of the demolition procedure, the infill walls in the 1st and 3rd floors were removed before the test. The infill walls were made of hollow clay tiles, which were in good condition. The net area of the clay tiles was about 1/2 of the gross area. The in-plane action of the infill walls contributes to the building stiffness and strength and affects the building response. Ignoring the effects of the infill walls and excluding them in the model would result in underestimating the building stiffness and strength.Using the SAP2000 computer program [8], two types of modeling for the infills are considered in this study: one uses two dimensional shell elements (Model A) and the other uses compressive struts (Model B) as suggested in FEMA356 [10] guidelines.4.1. Model A (infills modeled by shell elements)Infill walls are modeled with shell elements. However, the current version of the SAP2000 computer program includes only linear shell elements and cannot account for cracking. The tensile strength of the infill walls is set equal to 26 psi, with a modulus of elasticity of 644 ksi [10]. Because the formation ofcracks has a significant effect on the stiffness of the infill walls, the following iterative procedure is used to account for crack formation:(1) Assuming the infill walls are linear and uncracked, a nonlinear time history analysis is run. Note that plastic hinges exist in the beam elements and the segments of the beam elements where moment demand exceeds the cracking moment have a reduced moment of inertia.(2) The cracking pattern in the infill wall is determined by comparing stresses in the shells developed during the analysis with the tensile strength of infills.(3) Nodes are separated at the locations where tensile stress exceeds tensile strength. These steps are continued until the crack regions are properly modeled.4.2. Model B (infills modeled by struts)Infill walls are replaced with compressive struts as described in FEMA 356 [10] guidelines. Orientations of the struts are determined from the deformed shape of the structure after column removal and the location of openings.4.3. Column removalRemoval of the columns is simulated with the following procedure.(1) The structure is analyzed under the permanent loads and the internal forces are determined at the ends of the columns, which will be removed.(2) The model is modified by removing columns A2 and A3 on the first floor. Again the structure is statically analyzed under permanent loads. In this case, the internal forces at the ends of removed columns found in the first step are applied externally to the structure along with permanent loads. Note that the results of this analysis are identical to those of step 1.(3) The equal and opposite column end forces that were applied in the second step are dynamically imposed on the ends of the removed column within one millisecond [11] to simulate the removal of the columns, and dynamic analysis is conducted.4.4. Comparison of analytical and experimental resultsThe maximum calculated vertical displacement of the building occurs at joint A3 inthe second floor. Fig. 7 shows the experimental and analytical (Model A) vertical displacements of this joint (the AEM results will be discussed in the next section). Experimental data is obtained using the recordings of three potentiometers attached to joint A3 on one of their ends, and to the ground on the other ends. The peak displacements obtained experimentally and analytically (Model A) are 0.242 in (6.1 mm) and 0.252 in (6.4 mm), respectively, which differ only by about 4%. The experimental and analytical times corresponding to peak displacement are 0.069 s and 0.066 s, respectively. The analytical results show a permanent displacement of about 0.208 in (5.3 mm), which is about 14% smaller than the corresponding experimental value of 0.242 in (6.1 mm).Fig. 8 compares vertical displacement histories of joint A3 in the second floor estimated analytically based on Models A and B. As can be seen, modeling infills with struts (Model B) results in a maximum vertical displacement of joint A3 equal to about 0.45 in (11.4 mm), which is approximately 80% larger than the value obtained from Model A. Note that the results obtained from Model A are in close agreement with experimental results (see Fig. 7), while Model B significantly overestimates the deformation of the structure. If the maximum vertical displacement were larger, the infill walls were more severely cracked and the struts were more completely formed, the difference between the results of the two models (Models A and B) would be smaller.Fig. 9 compares the experimental and analytical (Model A) displacement of joint A2 in the second floor. Again, while the first peak vertical displacement obtained experimentally and analytically are in good agreement, the analytical permanent displacement under estimates the experimental value.Analytically estimated deformed shapes of the structure at the maximum vertical displacement based on Model A are shown in Fig. 10 with a magnification factor of 200. The experimentally measured deformed shape over the end regions of beamsA1–A2 and A3–B3 in the second floorare represented in the figure by solid lines. A total of 14 potentiometers were located at the top and bottom of the end regions of the second floor beams A1–A2 and A3–B3, which were the most critical elements in load redistribution. The beam top and corresponding bottom potentiometer recordings were used to calculate rotation between the sections where the potentiometer ends were connected. This was done by first finding the difference between the recorded deformations at the top and bottom of the beam, and then dividing the value by the distance (along the height of the beam section) between the two potentiometers. The expected deformed shapes between the measured end regions of the second floor beams are shown by dashed lines. As can be seen in the figures, analytically estimated deformed shapes of the beams are in good agreement with experimentally obtained deformed shapes.Analytical results of Model A show that only two plastic hinges are formed indicating rebar yielding. Also, four sections that did not have negative (top) reinforcement, reached cracking moment capacities and therefore cracked. Fig. 10 shows the locations of all the formed plastic hinges and cracks.。
土木工程毕业论文中英文翻译
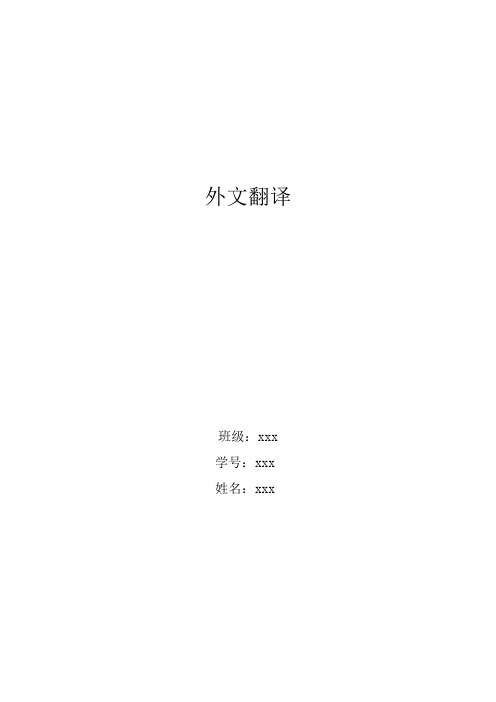
外文翻译班级:xxx 学号:xxx 姓名:xxx一、外文原文:Structural Systems to resist lateral loadsCommonly Used structural SystemsWith loads measured in tens of thousands kips, there is little room in the design of high-rise buildings for excessively complex thoughts. Indeed, the better high-rise buildings carry the universal traits of simplicity of thought and clarity of expression.It does not follow that there is no room for grand thoughts. Indeed, it is with such grand thoughts that the new family of high-rise buildings has evolved. Perhaps more important, the new concepts of but a few years ago have become commonplace in today’ s technology.Omitting some concepts that are related strictly to the materials of construction, the most commonly used structural systems used in high-rise buildings can be categorized as follows:1.Moment-resisting frames.2.Braced frames, including eccentrically braced frames.3.Shear walls, including steel plate shear walls.4.Tube-in-tube structures.5.Core-interactive structures.6.Cellular or bundled-tube systems.Particularly with the recent trend toward more complex forms, but in response also to the need for increased stiffness to resist the forces from wind and earthquake, most high-rise buildings have structural systems built up of combinations of frames, braced bents, shear walls, and related systems. Further, for the taller buildings, the majorities are composed of interactive elements in three-dimensional arrays.The method of combining these elements is the very essence of the design process for high-rise buildings. These combinations need evolve in response to environmental, functional, and cost considerations so as to provide efficient structures that provoke the architectural development to new heights. This is not to say that imaginative structural design can create great architecture. To the contrary, many examples of fine architecture have been created with only moderate support from the structural engineer, while only fine structure, not great architecture, can be developedwithout the genius and the leadership of a talented architect. In any event, the best of both is needed to formulate a truly extraordinary design of a high-rise building.While comprehensive discussions of these seven systems are generally available in the literature, further discussion is warranted here .The essence of the design process is distributed throughout the discussion.Moment-Resisting FramesPerhaps the most commonly used system in low-to medium-rise buildings, the moment-resisting frame, is characterized by linear horizontal and vertical members connected essentially rigidly at their joints. Such frames are used as a stand-alone system or in combination with other systems so as to provide the needed resistance to horizontal loads. In the taller of high-rise buildings, the system is likely to be found inappropriate for a stand-alone system, this because of the difficulty in mobilizing sufficient stiffness under lateral forces.Analysis can be accomplished by STRESS, STRUDL, or a host of other appropriate computer programs; analysis by the so-called portal method of the cantilever method has no place in today’s technology.Because of the intrinsic flexibility of the column/girder intersection, and because preliminary designs should aim to highlight weaknesses of systems, it is not unusual to use center-to-center dimensions for the frame in the preliminary analysis. Of course, in the latter phases of design, a realistic appraisal in-joint deformation is essential.Braced Frame sThe braced frame, intrinsically stiffer than the moment –resisting frame, finds also greater application to higher-rise buildings. The system is characterized by linear horizontal, vertical, and diagonal members, connected simply or rigidly at their joints. It is used commonly in conjunction with other systems for taller buildings and as a stand-alone system in low-to medium-rise buildings.While the use of structural steel in braced frames is common, concrete frames are more likely to be of the larger-scale variety.Of special interest in areas of high seismicity is the use of the eccentric braced frame.Again, analysis can be by STRESS, STRUDL, or any one of a series of two –or three dimensional analysis computer programs. And again, center-to-center dimensions are used commonly in the preliminary analysis.Shear wallsThe shear wall is yet another step forward along a progression of ever-stiffer structural systems. The system is characterized by relatively thin, generally (but not always) concrete elements that provide both structural strength and separation between building functions.In high-rise buildings, shear wall systems tend to have a relatively high aspect ratio, that is, their height tends to be large compared to their width. Lacking tension in the foundation system, any structural element is limited in its ability to resist overturning moment by the width of the system and by the gravity load supported by the element. Limited to a narrow overturning, One obvious use of the system, which does have the needed width, is in the exterior walls of building, where the requirement for windows is kept small.Structural steel shear walls, generally stiffened against buckling by a concrete overlay, have found application where shear loads are high. The system, intrinsically more economical than steel bracing, is particularly effective in carrying shear loads down through the taller floors in the areas immediately above grade. The system has the further advantage of having high ductility a feature of particular importance in areas of high seismicity.The analysis of shear wall systems is made complex because of the inevitable presence of large openings through these walls. Preliminary analysis can be by truss-analogy, by the finite element method, or by making use of a proprietary computer program designed to consider the interaction, or coupling, of shear walls.Framed or Braced TubesThe concept of the framed or braced or braced tube erupted into the technology with the IBM Building in Pittsburgh, but was followed immediately with the twin 110-story towers of the World Trade Center, New York and a number of other buildings .The system is characterized by three –dimensional frames, braced frames, or shear walls, forming a closed surface more or less cylindrical in nature, but of nearly any plan configuration. Because those columns that resistlateral forces are placed as far as possible from the cancroids of the system, the overall moment of inertia is increased and stiffness is very high.The analysis of tubular structures is done using three-dimensional concepts, or by two- dimensional analogy, where possible, whichever method is used, it must be capable of accounting for the effects of shear lag.The presence of shear lag, detected first in aircraft structures, is a serious limitation in the stiffness of framed tubes. The concept has limited recent applications of framed tubes to the shear of 60 stories. Designers have developed various techniques for reducing the effects of shear lag, most noticeably the use of belt trusses. This system finds application in buildings perhaps 40stories and higher. However, except for possible aesthetic considerations, belt trusses interfere with nearly every building function associated with the outside wall; the trusses are placed often at mechanical floors, mush to the disapproval of the designers of the mechanical systems. Nevertheless, as a cost-effective structural system, the belt truss works well and will likely find continued approval from designers. Numerous studies have sought to optimize the location of these trusses, with the optimum location very dependent on the number of trusses provided. Experience would indicate, however, that the location of these trusses is provided by the optimization of mechanical systems and by aesthetic considerations, as the economics of the structural system is not highly sensitive to belt truss location.Tube-in-Tube StructuresThe tubular framing system mobilizes every column in the exterior wall in resisting over-turning and shearing forces. The term‘tube-in-tube’is largely self-explanatory in that a second ring of columns, the ring surrounding the central service core of the building, is used as an inner framed or braced tube. The purpose of the second tube is to increase resistance to over turning and to increase lateral stiffness. The tubes need not be of the same character; that is, one tube could be framed, while the other could be braced.In considering this system, is important to understand clearly the difference between the shear and the flexural components of deflection, the terms being taken from beam analogy. In a framed tube, the shear component of deflection is associated with the bending deformation of columns and girders (i.e, the webs of the framed tube) while the flexural component is associated with the axial shortening and lengthening of columns (i.e, the flanges of the framed tube). In abraced tube, the shear component of deflection is associated with the axial deformation of diagonals while the flexural component of deflection is associated with the axial shortening and lengthening of columns.Following beam analogy, if plane surfaces remain plane (i.e, the floor slabs),then axial stresses in the columns of the outer tube, being farther form the neutral axis, will be substantially larger than the axial stresses in the inner tube. However, in the tube-in-tube design, when optimized, the axial stresses in the inner ring of columns may be as high, or even higher, than the axial stresses in the outer ring. This seeming anomaly is associated with differences in the shearing component of stiffness between the two systems. This is easiest to under-stand where the inner tube is conceived as a braced (i.e, shear-stiff) tube while the outer tube is conceived as a framed (i.e, shear-flexible) tube.Core Interactive StructuresCore interactive structures are a special case of a tube-in-tube wherein the two tubes are coupled together with some form of three-dimensional space frame. Indeed, the system is used often wherein the shear stiffness of the outer tube is zero. The United States Steel Building, Pittsburgh, illustrates the system very well. Here, the inner tube is a braced frame, the outer tube has no shear stiffness, and the two systems are coupled if they were considered as systems passing in a straight line from the “hat” structure. Note that the exterior columns would be improperly modeled if they were considered as systems passing in a straight line from the “hat” to the foundations; these columns are perhaps 15% stiffer as they follow the elastic curve of the braced core. Note also that the axial forces associated with the lateral forces in the inner columns change from tension to compression over the height of the tube, with the inflection point at about 5/8 of the height of the tube. The outer columns, of course, carry the same axial force under lateral load for the full height of the columns because the columns because the shear stiffness of the system is close to zero.The space structures of outrigger girders or trusses, that connect the inner tube to the outer tube, are located often at several levels in the building. The AT&T headquarters is an example of an astonishing array of interactive elements:1.The structural system is 94 ft (28.6m) wide, 196ft(59.7m) long, and 601ft (183.3m) high.2.Two inner tubes are provided, each 31ft(9.4m) by 40 ft (12.2m), centered 90 ft (27.4m)apart in the long direction of the building.3.The inner tubes are braced in the short direction, but with zero shear stiffness in the longdirection.4. A single outer tube is supplied, which encircles the building perimeter.5.The outer tube is a moment-resisting frame, but with zero shear stiffness for the center50ft(15.2m) of each of the long sides.6. A space-truss hat structure is provided at the top of the building.7. A similar space truss is located near the bottom of the building8.The entire assembly is laterally supported at the base on twin steel-plate tubes, because theshear stiffness of the outer tube goes to zero at the base of the building.Cellular structuresA classic example of a cellular structure is the Sears Tower, Chicago, a bundled tube structure of nine separate tubes. While the Sears Tower contains nine nearly identical tubes, the basic structural system has special application for buildings of irregular shape, as the several tubes need not be similar in plan shape, It is not uncommon that some of the individual tubes one of the strengths and one of the weaknesses of the system.This special weakness of this system, particularly in framed tubes, has to do with the concept of differential column shortening. The shortening of a column under load is given by the expression△=ΣfL/EFor buildings of 12 ft (3.66m) floor-to-floor distances and an average compressive stress of 15 ksi (138MPa), the shortening of a column under load is 15 (12)(12)/29,000 or 0.074in (1.9mm) per story. At 50 stories, the column will have shortened to 3.7 in. (94mm) less than its unstressed length. Where one cell of a bundled tube system is, say, 50stories high and an adjacent cell is, say, 100stories high, those columns near the boundary between .the two systems need to have this differential deflection reconciled.Major structural work has been found to be needed at such locations. In at least one building, the Rialto Project, Melbourne, the structural engineer found it necessary to vertically pre-stressthe lower height columns so as to reconcile the differential deflections of columns in close proximity with the post-tensioning of the shorter column simulating the weight to be added on to adjacent, higher columns.二、原文翻译:抗侧向荷载的结构体系常用的结构体系若已测出荷载量达数千万磅重,那么在高层建筑设计中就没有多少可以进行极其复杂的构思余地了。
土木工程外文文献及翻译
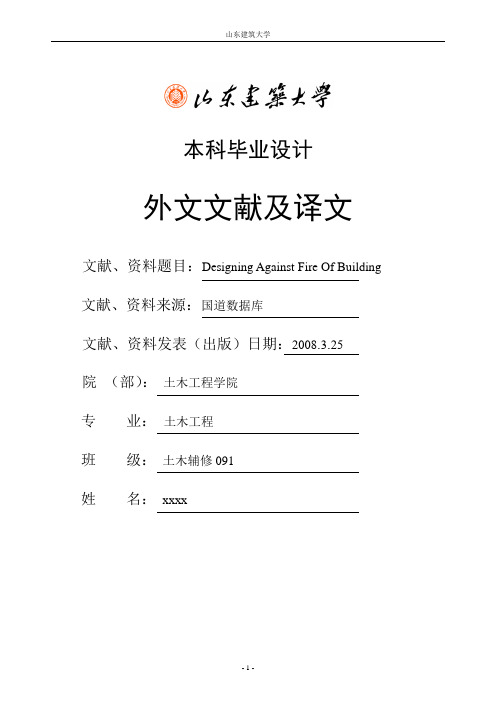
本科毕业设计外文文献及译文文献、资料题目:Designing Against Fire Of Building 文献、资料来源:国道数据库文献、资料发表(出版)日期:2008.3.25院(部):土木工程学院专业:土木工程班级:土木辅修091姓名:xxxx外文文献:Designing Against Fire Of BulidingxxxABSTRACT:This paper considers the design of buildings for fire safety. It is found that fire and the associ- ated effects on buildings is significantly different to other forms of loading such as gravity live loads, wind and earthquakes and their respective effects on the building structure. Fire events are derived from the human activities within buildings or from the malfunction of mechanical and electrical equipment provided within buildings to achieve a serviceable environment. It is therefore possible to directly influence the rate of fire starts within buildings by changing human behaviour, improved maintenance and improved design of mechanical and electrical systems. Furthermore, should a fire develops, it is possible to directly influence the resulting fire severity by the incorporation of fire safety systems such as sprinklers and to provide measures within the building to enable safer egress from the building. The ability to influence the rate of fire starts and the resulting fire severity is unique to the consideration of fire within buildings since other loads such as wind and earthquakes are directly a function of nature. The possible approaches for designing a building for fire safety are presented using an example of a multi-storey building constructed over a railway line. The design of both the transfer structure supporting the building over the railway and the levels above the transfer structure are considered in the context of current regulatory requirements. The principles and assumptions associ- ated with various approaches are discussed.1 INTRODUCTIONOther papers presented in this series consider the design of buildings for gravity loads, wind and earthquakes.The design of buildings against such load effects is to a large extent covered by engineering based standards referenced by the building regulations. This is not the case, to nearly the same extent, in the case of fire. Rather, it is building regulations such as the Building Code of Australia (BCA) that directly specify most of the requirements for fire safety of buildings with reference being made to Standards such as AS3600 or AS4100 for methods for determining the fire resistance of structural elements.The purpose of this paper is to consider the design of buildings for fire safety from an engineering perspective (as is currently done for other loads such as wind or earthquakes), whilst at the same time,putting such approaches in the context of the current regulatory requirements.At the outset,it needs to be noted that designing a building for fire safety is far morethan simply considering the building structure and whether it has sufficient structural adequacy.This is because fires can have a direct influence on occupants via smoke and heat and can grow in size and severity unlike other effects imposed on the building. Notwithstanding these comments, the focus of this paper will be largely on design issues associated with the building structure.Two situations associated with a building are used for the purpose of discussion. The multi-storey office building shown in Figure 1 is supported by a transfer structure that spans over a set of railway tracks. It is assumed that a wide range of rail traffic utilises these tracks including freight and diesel locomotives. The first situation to be considered from a fire safety perspective is the transfer structure.This is termed Situation 1 and the key questions are: what level of fire resistance is required for this transfer structure and how can this be determined? This situation has been chosen since it clearly falls outside the normal regulatory scope of most build- ing regulations. An engineering solution, rather than a prescriptive one is required. The second fire situation (termed Situation 2) corresponds to a fire within the office levels of the building and is covered by building regulations. This situation is chosen because it will enable a discussion of engineering approaches and how these interface with the building regulations–since both engineering and prescriptive solutions are possible.2 UNIQUENESS OF FIRE2.1 IntroductionWind and earthquakes can be considered to b e “natural” phenomena over which designers have no control except perhaps to choose the location of buildings more carefully on the basis of historical records and to design building to resist sufficiently high loads or accelerations for the particular location. Dead and live loads in buildings are the result of gravity. All of these loads are variable and it is possible (although generally unlikely) that the loads may exceed the resistance of the critical structural members resulting in structural failure.The nature and influence of fires in buildings are quite different to those associated with other“loads” to which a building may be subjected to. The essential differences are described in the following sections.2.2 Origin of FireIn most situations (ignoring bush fires), fire originates from human activities within the building or the malfunction of equipment placed within the building to provide a serviceable environment. It follows therefore that it is possible to influence the rate of fire starts by influencing human behaviour, limiting and monitoring human behaviour and improving thedesign of equipment and its maintenance. This is not the case for the usual loads applied to a building.2.3 Ability to InfluenceSince wind and earthquake are directly functions of nature, it is not possible to influence such events to any extent. One has to anticipate them and design accordingly. It may be possible to influence the level of live load in a building by conducting audits and placing restrictions on contents. However, in the case of a fire start, there are many factors that can be brought to bear to influence the ultimate size of the fire and its effect within the building. It is known that occupants within a building will often detect a fire and deal with it before it reaches a sig- nificant size. It is estimated that less than one fire in five (Favre, 1996) results in a call to the fire brigade and for fires reported to the fire brigade, the majority will be limited to the room of fire origin. In oc- cupied spaces, olfactory cues (smell) provide powerful evidence of the presence of even a small fire. The addition of a functional smoke detection system will further improve the likelihood of detection and of action being taken by the occupants.Fire fighting equipment, such as extinguishers and hose reels, is generally provided within buildings for the use of occupants and many organisations provide training for staff in respect of the use of such equipment.The growth of a fire can also be limited by automatic extinguishing systems such as sprinklers, which can be designed to have high levels of effectiveness.Fires can also be limited by the fire brigade depending on the size and location of the fire at the time of arrival. 2.4 Effects of FireThe structural elements in the vicinity of the fire will experience the effects of heat. The temperatures within the structural elements will increase with time of exposure to the fire, the rate of temperature rise being dictated by the thermal resistance of the structural element and the severity of the fire. The increase in temperatures within a member will result in both thermal expansion and,eventually,a reduction in the structural resistance of the member. Differential thermal expansion will lead to bowing of a member. Significant axial expansion will be accommodated in steel members by either overall or local buckling or yielding of local- ised regions. These effects will be detrimental for columns but for beams forming part of a floor system may assist in the development of other load resisting mechanisms (see Section 4.3.5).With the exception of the development of forces due to restraint of thermal expansion, fire does not impose loads on the structure but rather reduces stiffness and strength. Such effects are not instantaneous but are a function of time and this is different to the effects of loads such as earthquake and wind that are more or less instantaneous.Heating effects associated with a fire will not be significant or the rate of loss of capacity will be slowed if:(a) the fire is extinguished (e.g. an effective sprinkler system)(b) the fire is of insufficient severity – insufficient fuel, and/or(c)the structural elements have sufficient thermal mass and/or insulation to slow the rise in internal temperatureFire protection measures such as providing sufficient axis distance and dimensions for concrete elements, and sufficient insulation thickness for steel elements are examples of (c). These are illustrated in Figure 2.The two situations described in the introduction are now considered.3 FIRE WITHIN BUILDINGS3.1 Fire Safety ConsiderationsThe implications of fire within the occupied parts of the office building (Figure 1) (Situation 2) are now considered. Fire statistics for office buildings show that about one fatality is expected in an office building for every 1000 fires reported to the fire brigade. This is an order of magnitude less than the fatality rate associated with apartment buildings. More than two thirds of fires occur during occupied hours and this is due to the greater human activity and the greater use of services within the building. It is twice as likely that a fire that commences out of normal working hours will extend beyond the enclosure of fire origin.A relatively small fire can generate large quantities of smoke within the floor of fire origin. If the floor is of open-plan construction with few partitions, the presence of a fire during normal occupied hours is almost certain to be detected through the observation of smoke on the floor. The presence of full height partitions across the floor will slow the spread of smoke and possibly also the speed at which the occupants detect the fire. Any measures aimed at improving housekeeping, fire awareness and fire response will be beneficial in reducing thelikelihood of major fires during occupied hours.For multi-storey buildings, smoke detection systems and alarms are often provided to give “automatic” detection and warning to the occupants. An alarm signal is also transmitted to the fire brigade.Should the fire not be able to be controlled by the occupants on the fire floor, they will need to leave the floor of fire origin via the stairs. Stair enclosures may be designed to be fire-resistant but this may not be sufficient to keep the smoke out of the stairs. Many buildings incorporate stair pressurisation systems whereby positive airflow is introduced into the stairs upon detection of smoke within the building. However, this increases the forces required to open the stair doors and makes it increasingly difficult to access the stairs. It is quite likely that excessive door opening forces will exist(Fazio et al,2006)From a fire perspective, it is common to consider that a building consists of enclosures formed by the presence of walls and floors.An enclosure that has sufficiently fire-resistant boundaries (i.e. walls and floors) is considered to constitute a fire compartment and to be capable of limiting the spread of fire to an adjacent compartment. However, the ability of such boundaries to restrict the spread of fire can be severely limited by the need to provide natural lighting (windows)and access openings between the adjacent compartments (doors and stairs). Fire spread via the external openings (windows) is a distinct possibility given a fully developed fire. Limit- ing the window sizes and geometry can reduce but not eliminate the possibility of vertical fire spread.By far the most effective measure in limiting fire spread, other than the presence of occupants, is an effective sprinkler system that delivers water to a growing fire rapidly reducing the heat being generated and virtually extinguishing it.3.2 Estimating Fire SeverityIn the absence of measures to extinguish developing fires, or should such systems fail; severe fires can develop within buildings.In fire en gineering literature, the term “fire load” refers to the quantity of combustibles within an enclosure and not the loads (forces) applied to the structure during a fire. Similarly, fire load density refers to the quantity of fuel per unit area. It is normally expressed in terms of MJ/m2 or kg/m2 of wood equivalent. Surveys of combustibles for various occupancies (i.e offices, retail, hospitals, warehouses, etc)have been undertaken and a good summary of the available data is given in FCRC (1999). As would be expected, the fire load density is highly variable. Publications such as the International Fire Engineering Guidelines (2005) give fire load data in terms of the mean and 80th percentile.The latter level of fire load density is sometimes taken asthe characteristic fire load density and is sometimes taken as being distributed according to a Gumbel distribution (Schleich et al, 1999).The rate at which heat is released within an enclosure is termed the heat release rate (HRR) and normally expressed in megawatts (MW). The application of sufficient heat to a combustible material results in the generation of gases some of which are combustible. This process is called pyrolisation.Upon coming into contact with sufficient oxygen these gases ignite generating heat. The rate of burning(and therefore of heat generation) is therefore dependent on the flow of air to the gases generated by the pyrolising fuel.This flow is influenced by the shape of the enclosure (aspect ratio), and the position and size of any potential openings. It is found from experiments with single openings in approximately cubic enclosures that the rate of burning is directly proportional to A h where A is the area of the opening and h is the opening height. It is known that for deep enclosures with single openings that burning will occur initially closest to the opening moving back into the enclosure once the fuel closest to the opening is consumed (Thomas et al, 2005). Significant temperature variations throughout such enclosures can be expected.The use of the word ‘opening’ in relation to real building enclosures refers to any openings present around the walls including doors that are left open and any windows containing non fire-resistant glass.It is presumed that such glass breaks in the event of development of a significant fire. If the windows could be prevented from breaking and other sources of air to the enclosure limited, then the fire would be prevented from becoming a severe fire.Various methods have been developed for determining the potential severity of a fire within an enclosure.These are described in SFPE (2004). The predictions of these methods are variable and are mostly based on estimating a representative heat release rate (HRR) and the proportion of total fuel ςlikely to be consumed during the primary burning stage (Figure 4). Further studies of enclosure fires are required to assist with the development of improved models, as the behaviour is very complex.3.3 Role of the Building StructureIf the design objectives are to provide an adequate level of safety for the occupants and protection of adjacent properties from damage, then the structural adequacy of the building in fire need only be sufficient to allow the occupants to exit the building and for the building to ultimately deform in a way that does not lead to damage or fire spread to a building located on an adjacent site.These objectives are those associated with most building regulations includingthe Building Code of Australia (BCA). There could be other objectives including protection of the building against significant damage. In considering these various objectives, the following should be taken into account when considering the fire resistance of the building structure.3.3.1 Non-Structural ConsequencesSince fire can produce smoke and flame, it is important to ask whether these outcomes will threaten life safety within other parts of the building before the building is compromised by a loss of structural adequacy? Is search and rescue by the fire brigade not feasible given the likely extent of smoke? Will the loss of use of the building due to a severe fire result in major property and income loss? If the answer to these questions is in the affirmative, then it may be necessary to minimise the occurrence of a significant fire rather than simply assuming that the building structure needs to be designed for high levels of fire resistance. A low-rise shopping centre with levels interconnected by large voids is an example of such a situation.3.3.2 Other Fire Safety SystemsThe presence of other systems (e.g. sprinklers) within the building to minimise the occurrence of a serious fire can greatly reduce the need for the structural elements to have high levels of fire resistance. In this regard, the uncertainties of all fire-safety systems need to be considered. Irrespective of whether the fire safety system is the sprinkler system, stair pressurisation, compartmentation or the system giving the structure a fire-resistance level (e.g. concrete cover), there is an uncertainty of performance. Uncertainty data is available for sprinkler systems(because it is relatively easy to collect) but is not readily available for the other fire safety systems. This sometimes results in the designers and building regulators considering that only sprinkler systems are subject to uncertainty. In reality, it would appear that sprinklers systems have a high level of performance and can be designed to have very high levels of reliability.3.3.3 Height of BuildingIt takes longer for a tall building to be evacuated than a short building and therefore the structure of a tall building may need to have a higher level of fire resistance. The implications of collapse of tall buildings on adjacent properties are also greater than for buildings of only several storeys.3.3.4 Limited Extent of BurningIf the likely extent of burning is small in comparison with the plan area of the building, then the fire cannot have a significant impact on the overall stability of the building structure. Examples of situations where this is the case are open-deck carparks and very large area building such as shopping complexes where the fire-effected part is likely to be small in relation to area of the building floor plan.3.3.5 Behaviour of Floor ElementsThe effect of real fires on composite and concrete floors continues to be a subject of much research.Experimental testing at Cardington demonstrated that when parts of a composite floor are subject to heating, large displacement behaviour can develop that greatly assists the load carrying capacity of the floor beyond that which would predicted by considering only the behaviour of the beams and slabs in isolation.These situations have been analysed by both yield line methods that take into account the effects of membrane forces (Bailey, 2004) and finite element techniques. In essence, the methods illustrate that it is not necessary to insulate all structural steel elements in a composite floor to achieve high levels of fire resistance.This work also demonstrated that exposure of a composite floor having unprotected steel beams, to a localised fire, will not result in failure of the floor.A similar real fire test on a multistory reinforced concrete building demonstrated that the real structural behaviour in fire was significantly different to that expected using small displacement theory as for normal tempera- ture design (Bailey, 2002) with the performance being superior than that predicted by considering isolated member behaviour.3.4 Prescriptive Approach to DesignThe building regulations of most countries provide prescriptive requirements for the design of buildings for fire.These requirements are generally not subject to interpretation and compliance with them makes for simpler design approval–although not necessarily the most cost-effective designs.These provisions are often termed deemed-to-satisfy (DTS) provisions. All aspects of designing buildings for fire safety are covered–the provision of emergency exits, spacings between buildings, occupant fire fighting measures, detection and alarms, measures for automatic fire suppression, air and smoke handling requirements and last, but not least, requirements for compartmentation and fire resistance levels for structural members. However, there is little evidence that the requirements have been developed from a systematic evaluation of fire safety. Rather it would appear that many of the requirements have been added one to another to deal with another fire incident or to incorporate a new form of technology. There does not appear to have been any real attempt to determine which provision have the most significant influence on fire safety and whether some of the former provisions could be modified.The FRL requirements specified in the DTS provisions are traditionally considered to result in member resistances that will only rarely experience failure in the event of a fire.This is why it is acceptable to use the above arbitrary point in time load combination for assessing members in fire. There have been attempts to evaluate the various deemed-to-satisfy provisions (particularly the fire- resistance requirements)from a fire-engineering perspective taking intoaccount the possible variations in enclosure geometry, opening sizes and fire load (see FCRC, 1999).One of the outcomes of this evaluation was the recognition that deemed-to- satisfy provisions necessarily cover the broad range of buildings and thus must, on average, be quite onerous because of the magnitude of the above variations.It should be noted that the DTS provisions assume that compartmentation works and that fire is limited to a single compartment. This means that fire is normally only considered to exist at one level. Thus floors are assumed to be heated from below and columns only over one storey height.3.5 Performance-Based DesignAn approach that offers substantial benefits for individual buildings is the move towards performance-based regulations. This is permitted by regulations such as the BCA which state that a designer must demonstrate that the particular building will achieve the relevant performance requirements. The prescriptive provisions (i.e. the DTS provisions) are presumed to achieve these requirements. It is necessary to show that any building that does not conform to the DTS provisions will achieve the performance requirements.But what are the performance requirements? Most often the specified performance is simply a set of performance statements (such as with the Building Code of Australia)with no quantitative level given. Therefore, although these statements remind the designer of the key elements of design, they do not, in themselves, provide any measure against which to determine whether the design is adequately safe.Possible acceptance criteria are now considered.3.5.1 Acceptance CriteriaSome guidance as to the basis for acceptable designs is given in regulations such as the BCA. These and other possible bases are now considered in principle.(i)compare the levels of safety (with respect to achieving each of the design objectives) of the proposed alternative solution with those asso- ciated with a corresponding DTS solution for the building.This comparison may be done on either a qualitative or qualitative risk basis or perhaps a combination. In this case, the basis for comparison is an acceptable DTS solution. Such an approach requires a “holistic” approach to safety whereby all aspects relevant to safety, including the structure, are considered. This is, by far, the most common basis for acceptance.(ii)undertake a probabilistic risk assessment and show that the risk associated with the proposed design is less than that associated with common societal activities such as using pub lic transport. Undertaking a full probabilistic risk assessment can be very difficult for all but the simplest situations.Assuming that such an assessment is undertaken it will be necessary for the stakeholders to accept the nominated level of acceptable risk. Again, this requires a “holistic”approach to fire safety.(iii) a design is presented where it is demonstrated that all reasonable measures have been adopted to manage the risks and that any possible measures that have not been adopted will have negligible effect on the risk of not achieving the design objectives.(iv) as far as the building structure is concerned,benchmark the acceptable probability of failure in fire against that for normal temperature design. This is similar to the approach used when considering Building Situation 1 but only considers the building structure and not the effects of flame or smoke spread. It is not a holistic approach to fire safety.Finally, the questions of arson and terrorism must be considered. Deliberate acts of fire initiation range from relatively minor incidents to acts of mass destruction.Acts of arson are well within the accepted range of fire events experienced by build- ings(e.g. 8% of fire starts in offices are deemed "suspicious"). The simplest act is to use a small heat source to start a fire. The resulting fire will develop slowly in one location within the building and will most probably be controlled by the various fire- safety systems within the building. The outcome is likely to be the same even if an accelerant is used to assist fire spread.An important illustration of this occurred during the race riots in Los Angeles in 1992 (Hart 1992) when fires were started in many buildings often at multiple locations. In the case of buildings with sprinkler systems,the damage was limited and the fires significantly controlled.Although the intent was to destroy the buildings,the fire-safety systems were able to limit the resulting fires. Security measures are provided with systems such as sprinkler systems and include:- locking of valves- anti-tamper monitoring- location of valves in secure locationsFurthermore, access to significant buildings is often restricted by security measures.The very fact that the above steps have been taken demonstrates that acts of destruction within buildings are considered although most acts of arson do not involve any attempt to disable the fire-safety systems.At the one end of the spectrum is "simple" arson and at the other end, extremely rare acts where attempts are made to destroy the fire-safety systems along with substantial parts of the building.This can be only achieved through massive impact or the use of explosives. The latter may be achieved through explosives being introduced into the building or from outside by missile attack.The former could result from missile attack or from the collision of a large aircraft. The greater the destructiveness of the act,the greater the means and knowledge required. Conversely, the more extreme the act, the less confidence there can be in designing against suchan act. This is because the more extreme the event, the harder it is to predict precisely and the less understood will be its effects. The important point to recognise is that if sufficient means can be assembled, then it will always be possible to overcome a particular building design.Thus these acts are completely different to the other loadings to which a building is subjected such as wind,earthquake and gravity loading. This is because such acts of destruction are the work of intelligent beings and take into account the characteristics of the target.Should high-rise buildings be designed for given terrorist activities,then terrorists will simply use greater means to achieve the end result.For example, if buildings were designed to resist the impact effects from a certain size aircraft, then the use of a larger aircraft or more than one aircraft could still achieve destruction of the building. An appropriate strategy is therefore to minimise the likelihood of means of mass destruction getting into the hands of persons intent on such acts. This is not an engineering solution associated with the building structure.It should not be assumed that structural solutions are always the most appropriate, or indeed, possible.In the same way, aircrafts are not designed to survive a major fire or a crash landing but steps are taken to minimise the likelihood of either occurrence.The mobilization of large quantities of fire load (the normal combustibles on the floors) simultaneously on numerous levels throughout a building is well outside fire situations envisaged by current fire test standards and prescriptive regulations. Risk management measures to avoid such a possibility must be considered.4 CONCLUSIONSFire differs significantly from other “loads” such as wind, live load and earthquakes i n respect of its origin and its effects.Due to the fact that fire originates from human activities or equipment installed within buildings, it is possible to directly influence the potential effects on the building by reducing the rate of fire starts and providing measures to directly limit fire severity.The design of buildings for fire safety is mostly achieved by following the prescriptive requirements of building codes such as the BCA. For situations that fall outside of the scope of such regulations, or where proposed designs are not in accordance with the prescriptive requirements, it is possible to undertake performance-based fire engineering designs.However, there are no design codes or standards or detailed methodologies available for undertaking such designs.Building regulations require that such alternative designs satisfy performance requirements and give some guidance as to the basis for acceptance of these designs (i.e. acceptance criteria).This paper presents a number of possible acceptance criteria, all of which use the measure of risk level as the basis for comparison.Strictly, when considering the risks。
(完整版)土木工程专业英语课文原文及对照翻译
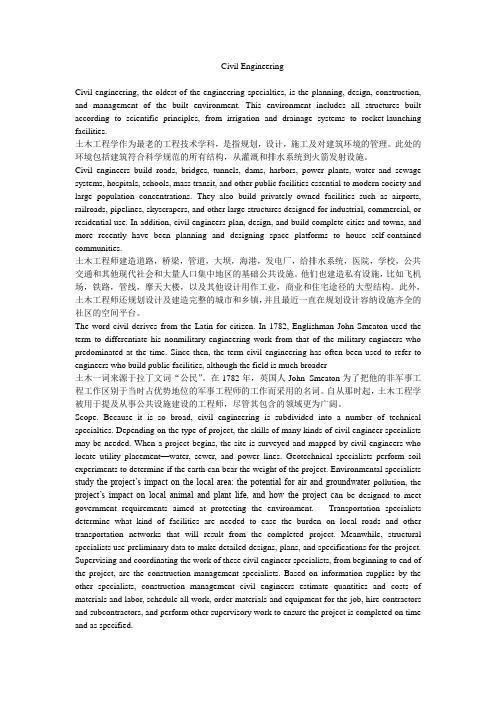
Civil EngineeringCivil engineering, the oldest of the engineering specialties, is the planning, design, construction, and management of the built environment. This environment includes all structures built according to scientific principles, from irrigation and drainage systems to rocket-launching facilities.土木工程学作为最老的工程技术学科,是指规划,设计,施工及对建筑环境的管理。
此处的环境包括建筑符合科学规范的所有结构,从灌溉和排水系统到火箭发射设施。
Civil engineers build roads, bridges, tunnels, dams, harbors, power plants, water and sewage systems, hospitals, schools, mass transit, and other public facilities essential to modern society and large population concentrations. They also build privately owned facilities such as airports, railroads, pipelines, skyscrapers, and other large structures designed for industrial, commercial, or residential use. In addition, civil engineers plan, design, and build complete cities and towns, and more recently have been planning and designing space platforms to house self-contained communities.土木工程师建造道路,桥梁,管道,大坝,海港,发电厂,给排水系统,医院,学校,公共交通和其他现代社会和大量人口集中地区的基础公共设施。
土木工程专业英语课文原文及对照翻译
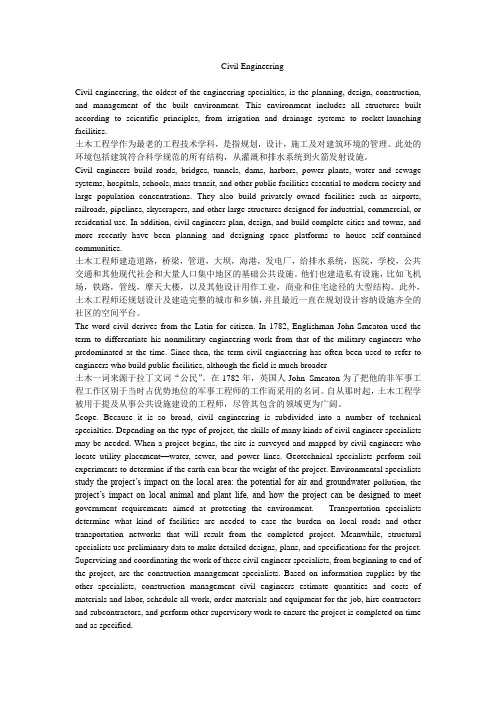
Civil EngineeringCivil engineering, the oldest of the engineering specialties, is the planning, design, construction, and management of the built environment. This environment includes all structures built according to scientific principles, from irrigation and drainage systems to rocket-launching facilities.土木工程学作为最老的工程技术学科,是指规划,设计,施工及对建筑环境的管理。
此处的环境包括建筑符合科学规范的所有结构,从灌溉和排水系统到火箭发射设施。
Civil engineers build roads, bridges, tunnels, dams, harbors, power plants, water and sewage systems, hospitals, schools, mass transit, and other public facilities essential to modern society and large population concentrations. They also build privately owned facilities such as airports, railroads, pipelines, skyscrapers, and other large structures designed for industrial, commercial, or residential use. In addition, civil engineers plan, design, and build complete cities and towns, and more recently have been planning and designing space platforms to house self-contained communities.土木工程师建造道路,桥梁,管道,大坝,海港,发电厂,给排水系统,医院,学校,公共交通和其他现代社会和大量人口集中地区的基础公共设施。
- 1、下载文档前请自行甄别文档内容的完整性,平台不提供额外的编辑、内容补充、找答案等附加服务。
- 2、"仅部分预览"的文档,不可在线预览部分如存在完整性等问题,可反馈申请退款(可完整预览的文档不适用该条件!)。
- 3、如文档侵犯您的权益,请联系客服反馈,我们会尽快为您处理(人工客服工作时间:9:00-18:30)。
A convection-conduction model for analysis of thefreeze-thawconditions in the surrounding rock wall of atunnel in permafrost regionsAbstractBased on the analyses of fundamental meteorological and hydrogeological conditions at the site of a tunnel in the cold regions, a combined convection-conduction model for air flow in the tunnel and temperature field in the surrounding has been constructed. Using the model, the air temperature distribution in the Xiluoqi No. 2 Tunnel has been simulated numerically. The simulated results are in agreement with the data observed. Then, based on the in situ conditions of sir temperature, atmospheric pressure, wind force, hydrogeology and engineering geology, the air-temperature relationship between the temperature on the surface of the tunnel wall and the air temperature at the entry and exit of the tunnel has been obtained, and the freeze-thaw conditions at the Dabanshan Tunnel which is now under construction is predicted.Keywords: tunnel in cold regions, convective heat exchange and conduction, freeze-thaw.A number of highway and railway tunnels have been constructed in the permafrost regions and their neighboring areas in China. Since the hydrological and thermal conditions changed after a tunnel was excavated,the surrounding wall rock materials often froze, the frost heaving caused damage to the liner layers and seeping water froze into ice diamonds,which seriously interfered with the communication and transportation. Similar problems of the freezing damage in the tunnels also appeared in other countries like Russia, Norway and Japan .Hence it is urgent to predict the freeze-thaw conditions in the surrounding rock materials and provide a basis for the design,construction andmaintenance of new tunnels in cold regions.Many tunnels,constructed in cold regions or their neighbouring areas,pass through the part beneath the permafrost base .After a tunnel is excavated,the original thermodynamical conditions in the surroundings are and thaw destroyed and replaced mainly by the air connections without the heat radiation, the conditions determined principally by the temperature and velocity of air flow in the tunnel,the coefficients of convective heat transfer on the tunnel wall,and the geothermal heat. In order to analyze and predict the freeze and thaw conditions of the surrounding wall rock of a tunnel,presuming the axial variations of air flow temperature and the coefficients of convective heat transfer, Lunardini discussed the freeze and thaw conditions by the approximate formulae obtained by Sham-sundar in study of freezing outside a circular tube with axial variations of coolant temperature .We simulated the temperature conditions on the surface of a tunnel wall varying similarly to the periodic changes of the outside air temperature .In fact,the temperatures of the air and the surrounding wall rock material affect each other so we cannot find the temperature variations of the air flow in advance; furthermore,it is difficult to quantify the coefficient of convective heat exchange at the surface of the tunnel wall .Therefore it is not practicable to define the temperature on the surface of the tunnel wall according to the outside air temperature .In this paper, we combine the air flow convective heat ex-change and heat conduction in the surrounding rock material into one model,and simulate the freeze-thaw conditions of the surrounding rock material based on the in situ conditions of air temperature,atmospheric pressure,wind force at the entry and exit of the tunnel,and the conditions of hydrogeology and engineering geology.Mathematical modelIn order to construct an appropriate model, we need the in situ fundamental conditions as a ba-sis .Here we use the conditions at the scene of the Dabanshan Tunnel. The Dabanshan Tunnel is lo-toted on the highway from Xining to Zhangye, south of the Datong River, at an elevation of 801.23 m, with a length of 1 530 m and an alignment from southwest to northeast. The tunnel runs from the southwest to thenortheast.Since the monthly-average air temperature is beneath 0`}C for eight months at the tunnel site each year and the construction would last for several years,the surrounding rock materials would become cooler during the construction .We conclude that, after excavation, the pattern of air flow would depend mainly on the dominant wind speed at the entry and exit,and the effects of the temperature difference between the inside and outside of the tunnel would be very small .Since the dominant wind direction is northeast at the tunnel site in winter, the air flow in the tunnel would go from the exit to the entry. Even though the dominant wind trend is southeastly in summer, considering the pressure difference, the temperature difference and the topography of the entry and exit,the air flow in the tunnel would also be from the exit to entry .Additionally,since the wind speed at the tunnel site is low,we could consider that the air flow would be principally laminar.Based on the reasons mentioned,we simplify the tunnel to a round tube,and consider that theair flow and temperature are symmetrical about the axis of the tunnel,Ignoring the influence of the air temperature on the speed of air flow, we obtain the following equation:where t ,x ,r are the time ,axial and radial coordinates; U ,V are axial and radial wind speeds; T is temperature; p is the effective pressure(that is ,air pressure divided by air density); v is the kinematic viscosity of air; a is the thermal conductivity of air; L is the length of the tunnel; R is the equivalent radius of the tunnel section; D is the length of time after the tunnel construction;,f S (t), u S (t) are frozen and thawed parts in the surrounding rock materials respectively; f λ,u λand f C ,u C are thermal conductivities and volumetric thermal capacities in frozen and thawed parts respectively; X= (x , r),ξ(t) is phase change front; Lh is heat latent of freezing water; and To is critical freezing temperature of rock ( here we assume To= -0.1℃).2 used for solving the modelEquation(1)shows flow. We first solve those concerning temperature at that the temperature of the surrounding rock does not affect the speed of air equations concerning the speed of air flow, and then solve those equations every time elapse.2. 1 Procedure used for solving the continuity and momentum equations Since the first three equations in(1) are not independent we derive the second equation by xand the third equation by r. After preliminary calculation we obtain the following elliptic equation concerning the effective pressure p:Then we solve equations in(1) using the following procedures:(i ) Assume the values for U0,V0;( ii ) substituting U0,V0 into eq. (2),and solving (2),we obtain p0;(iii) solving the first and second equations of(1),we obtain U0,V1;(iv) solving the first and third equations of(1),we obtain U2,V2;(v) calculating the momentum-average of U1,v1 and U2,v2,we obtain the new U0,V0;then return to (ii);(vi) iterating as above until the disparity of those solutions in two consecutive iterations is sufficiently small or is satisfied ,we then take those values of p0,U0 and V0 as the initial values for the next elapse and solve those equations concerning the temperature..2 .2 Entire method used for solving the energy equationsAs mentioned previously ,the temperature field of the surrounding rock and the air flow affect each other. Thus the surface of the tunnel wall is both the boundary of the temperature field in the surrounding rock and the boundary of the temperature field in air flow .Therefore , it is difficult to separately identify the temperature on the tunnel wall surface ,and we cannot independently solve those equations concerning the temperature of air flow and those equations concerning the temperature of the surrounding rock .In order to cope with this problem ,we simultaneously solve the two groups of equations based on the fact that at the tunnel wall surface both temperatures are equal .We should bear in mind the phase change while solving those equations concerning the temperature of the surrounding rock ,and the convection while solving those equations concerning the temperature of the air flow, and we only need to smooth those relative parameters at the tunnel wall surface .The solving methods for the equations with the phase change are the same as in reference [3].2.3 Determination of thermal parameters and initial and boundaryconditions2.3.1 Determination of the thermal parameters. Using p= H ,we calculateair pressure p at elevation H and calculate the air density ρ using formula GTP =ρ, where T is the yearly-average absolute air temperature ,and G is the humidity constant of air. Letting P C be the thermal capacity with fixed pressure, λ the thermal conductivity ,and μ the dynamic viscosity of air flow, we calculate the thermal conductivity and kinematic viscosity using the formulas ρλP C =a and ρμν=. The thermal parameters of the surrounding rock are determined from the tunnel site.2 .3.2 Determination of the initial and boundary conditions .Choose the observed monthly average wind speed at the entry and exit as boundary conditions of wind speed ,and choose the relative effective pressure p=0 at the exit ( that is ,the entry of the dominant wind trend) and ]5[22/)/1(v d kL p ⨯+= on the section of entry ( that is ,the exit of the dominant wind trend ),where k is the coefficient of resistance along the tunnel wall, d = 2R ,and v is the axial average speed. We approximate T varying by the sine law according to the data observed at the scene and provide a suitable boundary value based on the position of the permafrost base and the geothermal gradient of the thaw rock materials beneath the permafrost base.3 A simulated exampleUsing the model and the solving method mentioned above ,we simulate the varying law of the air temperature in the tunnel along with the temperature at the entry and exit of the Xiluoqi Tunnel .We observe that the simulated results are close to the data observed[6].The Xiluoqi No .2 Tunnel is located on the Nongling railway in northeastern China and passes through the part beneath the permafrost base .It has a length of 1 160 m running from the northwest to the southeast, with the entry of the tunnel in the northwest ,and the elevation is about 700 m. The dominant wind direction in the tunnel is from northwest to southeast, with a maximum monthly-average speed of 3 m/s and a minimum monthly-average speed of 1 .7 m/s . Based on the data observed ,weapproximate the varying sine law of air temperature at the entry and exit with yearly averages of -5℃,-6.4℃ and amplitudes of 18.9℃ and 17.6℃respectively. The equivalent diameter is 5 .8m,and the resistant coefficient along the tunnel wall is the effect of the thermal parameter of the surrounding rock on the air flow is much smaller than that of wind speed,pressure and temperature at the entry and exit,we refer to the data observed in the Dabanshan Tunnel for the thermal parameters.Figure 1 shows the simulated yearly-average air temperature inside and at the entry and exit of the tunnel compared with the data observed .We observe that the difference is less than 0 .2 `C from the entry to exit.Figure 2 shows a comparison of the simulated and observed monthly-average air temperature in-side (distance greater than 100 m from the entry and exit) the tunnel. We observe that the principal law is almost the same,and the main reason for the difference is the errors that came from approximating the varying sine law at the entry and exit; especially , the maximum monthly-average air temperature of 1979 was not for July but for August.. Comparison of simulated and observed air temperature in Xiluoqi Tunnel in ,simulated values;2,observed valuescomparison of simulated and observed air temperature insideThe Xiluoqi Tunnel in ,simulated values;2,observed values4 Prediction of the freeze-thaw conditions for the Dabanshan Tunnel 4 .1 Thermal parameter and initial and boundary conditionsUsing the elevation of 3 800 m and the yearly-average air temperature of -3℃, we calculate the air density p=0 .774 kg/3 steam exists In the air, we choose the thermal capacity with a fixed pressure of air ),./(8744.10C kg kJ C p = heat conductivity )./(100.202C m W -⨯=λ andand the dynamic viscosity )../(10218.96s m kg -⨯=μ After calculation we obtain the thermal diffusivity a= 1 .3788s m /1025-⨯ and the kinematic viscosity ,s m /1019.125-⨯=ν .Considering that the section of automobiles is much smaller than that of the tunnel and the auto-mobiles pass through the tunnel at a low speed ,we ignore the piston effects ,coming from the movement of automobiles ,in the diffusion of the air.We consider the rock as a whole component and choose the dry volumetric cavity 3/2400m kg d =λ,content of water and unfrozen water W=3% and W=1%, and the thermal conductivity c m W o u ./9.1=λ,c m W o f ./0.2=λ,heat capacityc kg kJ C o V ./8.0= and d u f W w C γ⨯++=1)128.48.0(,d u u Ww C γ⨯++=1)128.48.0( According to the data observed at the tunnel site ,the maximum monthly-average wind speed is about 3 .5 m/s ,and the minimum monthly-average wind speed is about 2 .5 m/s .We approximate the wind speed at the entry and exit as )/](5.2)7(028.0[)(2s m t t v +-⨯=, where t is in month. The initial wind speed in the tunnel is set to be.0),,0(),)(1(),,0(2=-=r x V R r U r x U a The initial and boundary values of temperature T are set to bewhere f(x) is the distance from the vault to the permafrost base ,andR0=25 m is the radius of do-main of solution T. We assume that the geothermal gradient is 3%,the yearly-average air temperature outside tunnel the is A=-3C 0,and the amplitude is B=12C 0.As for the boundary of R=Ro ,we first solve the equations considering R=Ro as the first type of boundary; that is we assume that T=f(x) 3%C 0on R=Ro. We find that, after one year, the heat flow trend will have changed in the range of radius between 5 and 25m in the surrounding rock.. Considering that the rock will be cooler hereafter and it will be affected yet by geothermal heat, we appoximately assume that the boundary R=Ro is the second type of boundary; that is ,we assume that the gradient value ,obtained from the calculation up to the end of the first year after excavation under the first type of boundary value, is the gradient on R=Ro of T.Considering the surrounding rock to be cooler during the period of construction ,we calculatefrom January and iterate some elapses of time under the same boundary. Then we let the boundaryvalues vary and solve the equations step by step(it can be proved that the solution will not depend on the choice of initial values after many time elapses ).1)The yearly-average temperature on the surface wall of the tunnel is approximately equal to the ai4 .2 Calculated resultsFigures 3 and 4 show the variations of the monthly-average temperatures on the surface of the tunnel wall along with the variations at the entry and exit .Figs .5 and 6 show the year when permafrost begins to form and the maximum thawed depth after permafrost formed in different surrounding sections.monthly-average temperature of the monthly-On the surface of Dabanshan , average temperature on the surface The month,I=1,2,3,,,12 tunnel with that outside the tunnel.1,inner temperature on the surface ;2,outside air temperatureyear when permafrost maximum thawed depth afterBegins to from in different permafrost formed in different years Sections of the surroundingrock4 .3 Preliminary conclusionBased on the initial-boundary conditions and thermal parameters mentioned above, we obtain the following preliminary conclusions: r temperature at the entry and exit. It is warmer during the cold season and cooler during the warm season in the internal part (more than 100 m from the entry and exit) of the tunnel than at the entry and exit . Fig .1 shows that the internal monthly-average temperature on the surface of the tunnel wall is 1.2℃ higher in January, February and December, 1℃higher in March and October, and 1 .6℃ lower in June and August, and 2qC lower in July than the air temperature at the entry and exit. In othermonths the infernal temperature on the surface of the tunnel wall approximately equals the air temperature at the entry and exit.2) Since it is affected by the geothermal heat in the internal surrounding section,especially in the central part, the internal amplitude of the yearly-average temperature on the surface of the tunnel wall decreases and is 1 .6℃ lower than that at the entry and exit.3 ) Under the conditions that the surrounding rock is compact , without a great amount of under-ground water, and using a thermal insulating layer(as designed PU with depth of 0.05 m and heat conductivity λ= W/m℃,FBT with depth of 0.085 m and heat conductivity λ=m℃),in the third year after tunnel construction,the surrounding rock will begin to form permafrost in the range of 200 m from the entry and exit .In the first and the second year after construction, the surrounding rock will begin to form permafrost in the range of 40 and 100m from the entry and exit respectively .In the central part,more than 200m from the entry and exit, permafrost will begin to form in the eighth year. Near the center of the tunnel,permafrost will appear in the 14-15th years. During the first and second years after permafrost formed,the maximum of annual thawed depth is large (especially in the central part of the surrounding rock section) and thereafter it decreases every year. The maximum of annual thawed depth will be stable until the 19-20th years and will remain in s range of 2-3 m.4) If permafrost forms entirely in the surrounding rock,the permafrost will provide a water-isolating layer and be favourable for communication and transportation .However, in the process of construction,we found a lot of underground water in some sections of the surrounding rock .It will permanently exist in those sections,seeping out water and resulting in freezing damage to the liner layer. Further work will be reported elsewhere.严寒地区隧道围岩冻融状况分析的导热与对流换热模型摘要通过对严寒地区隧道现场基本气象条件的分析,建立了隧道内空气与围岩对流换热及固体导热的综合模型;用此模型对大兴安岭西罗奇2号隧道的洞内气温分布进行了模拟计算,结果与实测值基本一致;分析预报了正在开凿的祁连山区大坂山隧道开通运营后洞内温度及围岩冻结、融化状况.关键词严寒地区隧道导热与对流换热冻结与融化在我国多年冻土分布及邻近地区,修筑了公路和铁路隧道几十座.由于隧道开通后洞内水热条件的变化;,普遍引起洞内围岩冻结,造成对衬砌层的冻胀破坏以及洞内渗水冻结成冰凌等,严重影响了正常交通.类似隧道冻害问题同样出现在其他国家(苏联、挪威、日本等)的寒冷地区.如何预测分析隧道开挖后围岩的冻结状况,为严寒地区隧道建设的设计、施工及维护提供依据,这是一个亟待解决的重要课题.在多年冻土及其临近地区修筑的隧道,多数除进出口部分外从多年冻土下限以下岩层穿过.隧道贯通后,围岩内原有的稳定热力学条件遭到破坏,代之以阻断热辐射、开放通风对流为特征的新的热力系统.隧道开通运营后,围岩的冻融特性将主要由流经洞内的气流的温度、速度、气—固交界面的换热以及地热梯度所确定.为分析预测隧道开通后围岩的冻融特性,Lu-nardini借用Shamsundar研究圆形制冷管周围土体冻融特性时所得的近似公式,讨论过围岩的冻融特性.我们也曾就壁面温度随气温周期性变化的情况,分析计算了隧道围岩的温度场[3].但实际情况下,围岩与气体的温度场相互作用,隧道内气体温度的变化规律无法预先知道,加之洞壁表面的换热系数在技术上很难测定,从而由气温的变化确定壁面温度的变化难以实现.本文通过气一固祸合的办法,把气体、固体的换热和导热作为整体来处理,从洞口气温、风速和空气湿度、压力及围岩的水热物理参数等基本数据出发,计算出围岩的温度场.1数学模型为确定合适的数学模型,须以现场的基本情况为依据.这里我们以青海祁连山区大坂山公路隧道的基本情况为背景来加以说明.大坂山隧道位于西宁一张业公路大河以南,海拔~3801.23 m,全长1530 m ,隧道近西南—东北走向.由于大坂山地区隧道施工现场平均气温为负温的时间每年约长8个月,加之施工时间持续数年,围岩在施土过程中己经预冷,所以隧道开通运营后,洞内气体流动的形态主要由进出口的主导风速所确定,而受洞内围岩地温与洞外气温的温度压差的影响较小;冬季祁连山区盛行西北风,气流将从隧道出曰流向进口端,夏季虽然祁连山区盛行东偏南风,但考虑到洞口两端气压差、温度压差以及进出口地形等因素,洞内气流仍将由出口北端流向进口端.另外,由于现场年平均风速不大,可以认为洞内气体将以层流为主基于以上基本情况,我们将隧道简化成圆筒,并认为气流、温度等关十隧道中心线轴对称,忽略气体温度的变化对其流速的影响,可有如下的方程:其中t 为时间,x 为轴向坐标,r 为径向坐标;U, V 分别为轴向和径向速度,T 为温度,P 为有效压力(即空气压力与空气密度之比少,V 为空气运动粘性系数,a 为空气的导温系数,L 为隧道长度,R 为隧道的当量半径,D 为时间长度)(t S f , )(t S u 分别为围岩的冻、融区域. f λ,u λ分别为冻、融状态下的热传导系数,f C ,u C 分别为冻、融状态下的体积热容量,X=(x,r) , )(t ξ为冻、融相变界面,To 为岩石冻结临界温度(这里具体计算时取To=C 0,h L 为水的相变潜热.2 求解过程由方程(1)知,围岩的温度的高低不影响气体的流动速度,所以我们可先解出速度,再解温度.连续性方程和动量方程的求解由于方程((1)的前3个方程不是相互独立的,通过将动量方程分别对x 和r 求导,经整理化简,我们得到关于压力P 的如下椭圆型方程:于是,对方程(1)中的连续性方程和动量方程的求解,我们按如下步骤进行:(1)设定速度0U ,0V ;( 2)将0U ,0V 代入方程并求解,得0P(3)联立方程(1)的第一个和第二个方程,解得一组解1U ,1V ;(4)联立方程((1)的第一个和第三个方程,解得一组解2U ,2V ;(5)对((3) ,(4)得到的速度进行动量平均,得新的0U ,0V 返回(2) ;(6)按上述方法进行迭代,直到前后两次的速度值之差足够小.以0P ,0U ,0V 作为本时段的解,下一时段求解时以此作为迭代初值.2. 2 能量方程的整体解法如前所述,围岩与空气的温度场相互作用,壁面既是气体温度场的边界,又是固体温度场的边界,壁面的温度值难以确定,我们无法分别独立地求解隧道内的气体温度场和围岩温度场.为克服这一困难,我们利用在洞壁表面上,固体温度等于气体温度这一事实,把隧道内气体的温度和围岩内固体的温度放在一起求解,这样壁面温度将作为末知量被解出来.只是需要注意两点:解流体温度场时不考虑相变和解固体温度时没有对流项;在洞壁表面上方程系数的光滑化.另外,带相变的温度场的算法与文献[3]相同.2. 3热参数及初边值的确定热参数的确定方法: 用p=计算出海拔高度为H 的隧道现场的大气 压强,再由GTP =ρ计算出现场空气密度ρ,其中T 为现场大气的年平均绝对温度,G 为空气的气体常数.记定压比热为P C ,导热系数为λ,空气的动力粘性系数为μ.按ρλP C =a 和ρμν= 计算空气的导温系数和运动粘性系数.围岩的热物理参数则由现场采样测定.初边值的确定方法:洞曰风速取为现场观测的各月平均风速.取卞导风进曰的相对有效气压为0,主导风出口的气压则取为]5[22/)/1(v d kL p ⨯+=,这里k 为隧道内的沿程阻力系数,L 为隧道长度,d 为隧道端面的当量直径,ν为进口端面轴向平均速度.进出口气温年变化规律由现场观测资料,用正弦曲线拟合,围岩内计算区域的边界按现场多年冻土下限和地热梯度确定出适当的温度值或温度梯度. 3 计算实例按以上所述的模型及计算方法,我们对大兴安岭西罗奇2号隧道内气温随洞曰外气温变化的规律进行了模拟计算验证,所得结果与实测值[6]相比较,基本规律一致.西罗奇2号隧道是位十东北嫩林线的一座非多年冻土单线铁路隧道,全长1160 m ,隧道近西北一东南向,高洞口位于西北向,冬季隧道主导风向为西北风.洞口海拔高度约为700 m ,月平均最高风速约为3m/s,最低风速约为1.7m/s.根据现场观测资料,我们将进出口气温拟合为年平均分别为-5C 0和C 0,年变化振幅分别为C 0和C 0的正弦曲线.隧道的当量直径为5.8 m,沿程阻力系数取为.由于围岩的热物理参数对计算洞内气温的影响远比洞口的风速、压力及气温的影响小得多,我们这里参考使用了大坂山隧道的资料.图1给出了洞口及洞内年平均气温的计算值与观测值比较的情况,从进口到出口,两值之差都小于C 0.图2给出了洞内 (距进出口l00m 以上)月平均气温的计算值与观测值比较的情况,可以看出温度变化的基本规律完全一致,造成两值之差的主要原因是洞口气温年变化规律之正弦曲线的拟合误差,特别是1979年隧道现场月平均最高气温不是在7月份,而是在8月份.图1. 比较1979年在西罗奇周家山2号隧道仿真试验与观察的空气温度.1、模拟值;2、观测值图2。