seasonal variations and spatial distribution of carbonaceous aerosols in Taiwan
英语季节课件ppt
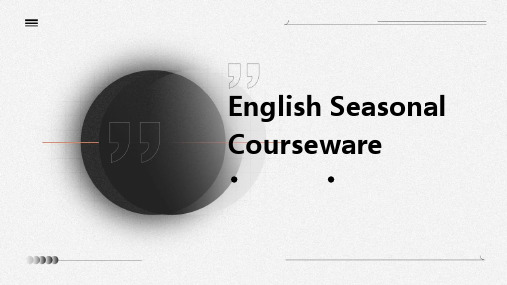
Beach parties are a popular summer activity, with people gathering to enjoy the sun, sand, and sea
Fireworks Display
Summer of bringing fireworks displays to cellular special occasions or as part of cultural festivals
The air is critical and fresh, perfect for a walk in the park
I love the sound of clutch leaves under my feet as I walk through the woods
Introduction to Autumn Activities and Customers
Introduction to Spring Activities and Customers
Eastern
Spring Break
A Christian holiday celebrating the recursion of Jesus Christ, often associated with egg hunts, bunny rabbits, and colored Easter eggs
03
"Watermelon," "Strawberry," "corn," "tomato"
Describe the scene of summer presence patterns
描述气候英语作文
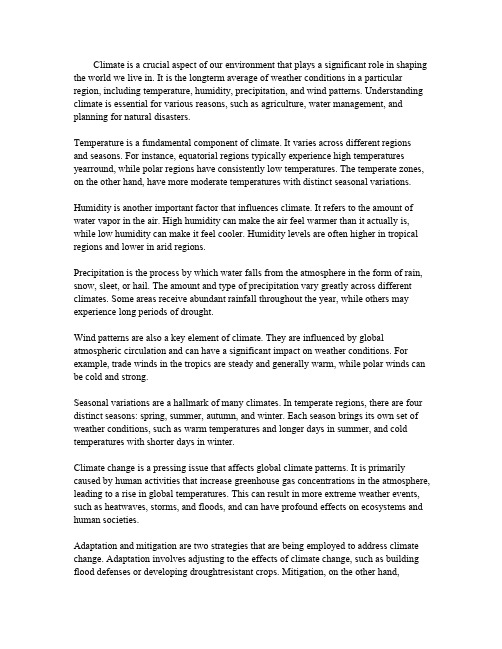
Climate is a crucial aspect of our environment that plays a significant role in shaping the world we live in.It is the longterm average of weather conditions in a particular region,including temperature,humidity,precipitation,and wind patterns.Understanding climate is essential for various reasons,such as agriculture,water management,and planning for natural disasters.Temperature is a fundamental component of climate.It varies across different regions and seasons.For instance,equatorial regions typically experience high temperatures yearround,while polar regions have consistently low temperatures.The temperate zones, on the other hand,have more moderate temperatures with distinct seasonal variations.Humidity is another important factor that influences climate.It refers to the amount of water vapor in the air.High humidity can make the air feel warmer than it actually is, while low humidity can make it feel cooler.Humidity levels are often higher in tropical regions and lower in arid regions.Precipitation is the process by which water falls from the atmosphere in the form of rain, snow,sleet,or hail.The amount and type of precipitation vary greatly across different climates.Some areas receive abundant rainfall throughout the year,while others may experience long periods of drought.Wind patterns are also a key element of climate.They are influenced by global atmospheric circulation and can have a significant impact on weather conditions.For example,trade winds in the tropics are steady and generally warm,while polar winds can be cold and strong.Seasonal variations are a hallmark of many climates.In temperate regions,there are four distinct seasons:spring,summer,autumn,and winter.Each season brings its own set of weather conditions,such as warm temperatures and longer days in summer,and cold temperatures with shorter days in winter.Climate change is a pressing issue that affects global climate patterns.It is primarily caused by human activities that increase greenhouse gas concentrations in the atmosphere, leading to a rise in global temperatures.This can result in more extreme weather events, such as heatwaves,storms,and floods,and can have profound effects on ecosystems and human societies.Adaptation and mitigation are two strategies that are being employed to address climate change.Adaptation involves adjusting to the effects of climate change,such as building flood defenses or developing droughtresistant crops.Mitigation,on the other hand,focuses on reducing greenhouse gas emissions to slow the rate of climate change.In conclusion,climate is a multifaceted phenomenon that influences every aspect of life on Earth.Understanding its patterns and changes is essential for the sustainable management of our environment and for ensuring the wellbeing of current and future generations.。
Seasonal dynamics of ciliated protozoa and their potential food in an eutrophic estuary
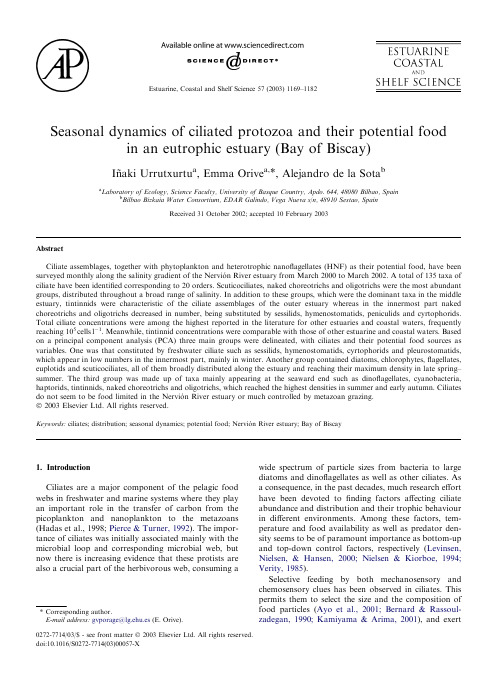
Seasonal dynamics of ciliated protozoa and their potential foodin an eutrophic estuary (Bay of Biscay)In ~aki Urrutxurtu a ,Emma Orive a,*,Alejandro de la Sota baLaboratory of Ecology,Science Faculty,University of Basque Country,Apdo.644,48080Bilbao,Spain bBilbao Bizkaia Water Consortium,EDAR Galindo,Vega Nueva s/n,48910Sestao,SpainReceived 31October 2002;accepted 10February 2003AbstractCiliate assemblages,together with phytoplankton and heterotrophic nanoflagellates (HNF)as their potential food,have beensurveyed monthly along the salinity gradient of the Nervion River estuary from March 2000to March 2002.A total of 135taxa of ciliate have been identified corresponding to 20orders.Scuticociliates,naked choreotrichs and oligotrichs were the most abundant groups,distributed throughout a broad range of salinity.In addition to these groups,which were the dominant taxa in the middle estuary,tintinnids were characteristic of the ciliate assemblages of the outer estuary whereas in the innermost part naked choreotrichs and oligotrichs decreased in number,being substituted by sessilids,hymenostomatids,peniculids and cyrtophorids.Total ciliate concentrations were among the highest reported in the literature for other estuaries and coastal waters,frequently reaching 105cells l ÿ1.Meanwhile,tintinnid concentrations were comparable with those of other estuarine and coastal waters.Based on a principal component analysis (PCA)three main groups were delineated,with ciliates and their potential food sources as variables.One was that constituted by freshwater ciliate such as sessilids,hymenostomatids,cyrtophorids and pleurostomatids,which appear in low numbers in the innermost part,mainly in winter.Another group contained diatoms,chlorophytes,flagellates,euplotids and scuticociliates,all of them broadly distributed along the estuary and reaching their maximum density in late spring–summer.The third group was made up of taxa mainly appearing at the seaward end such as dinoflagellates,cyanobacteria,haptorids,tintinnids,naked choreotrichs and oligotrichs,which reached the highest densities in summer and early autumn.Ciliatesdo not seem to be food limited in the Nervion River estuary or much controlled by metazoan grazing.Ó2003Elsevier Ltd.All rights reserved.Keywords:ciliates;distribution;seasonal dynamics;potential food;Nervion River estuary;Bay of Biscay 1.IntroductionCiliates are a major component of the pelagic foodwebs in freshwater and marine systems where they play an important role in the transfer of carbon from the picoplankton and nanoplankton to the metazoans (Hadas et al.,1998;Pierce &Turner,1992).The impor-tance of ciliates was initially associated mainly with the microbial loop and corresponding microbial web,but now there is increasing evidence that these protists are also a crucial part of the herbivorous web,consuming awide spectrum of particle sizes from bacteria to large diatoms and dinoflagellates as well as other ciliates.As a consequence,in the past decades,much research effort have been devoted to finding factors affecting ciliate abundance and distribution and their trophic behaviour in different environments.Among these factors,tem-perature and food availability as well as predator den-sity seems to be of paramount importance as bottom-up and top-down control factors,respectively (Levinsen,Nielsen,&Hansen,2000;Nielsen &Kiorboe,1994;Verity,1985).Selective feeding by both mechanosensory and chemosensory clues has been observed in ciliates.This permits them to select the size and the composition of food particles (Ayo et al.,2001;Bernard &Rassoul-zadegan,1990;Kamiyama &Arima,2001),and exert*Corresponding author.E-mail address:gvporage@lg.ehu.es (E.Orive).Estuarine,Coastal and Shelf Science 57(2003)1169–11820272-7714/03/$-see front matter Ó2003Elsevier Ltd.All rights reserved.doi:10.1016/S0272-7714(03)00057-Xa strong influence on the dynamics of the other com-ponents of the microplankton.Estuaries are known to contain higher concentrations of nutrients and organics than most aquatic ecosystems. Although estuaries have generally lower specific di-versity than their adjacent marine or freshwater rela-tives,planktonic euryhaline species both autotrophic and heterotrophic forms may grow in these rich systems to bloom proportions when unchecked by other control-ling factors(Cloern,2001).The Nervio n River is a nutrient rich estuary,that receives large amounts of dissolved and particulate organic matter from the catchment basin and from a wastewater treatment plant(WTP).Recently,the water quality has experienced a remarkable improvement lead-ing to increased water transparency that has permitted the development and growth of the phytoplankton. Meanwhile,the estuary presents features of eutrophic waters with high levels of ammonia and low oxygen concentration together with high concentrations of bacteria and organics(Bilbao-Biscay Water Consortium, personal communication).On the other hand,recent studies on the sediment have revealed the existence of an impoverished invertebrate fauna,reduced to a few oligochaeta(Gonza lez-Oreja,personal communication). Besides,rotifers,cladocera and copepods,which are major consumers on ciliate,are almost absent from the estuary(Uriarte,personal communication).The aim of this paper was to know the relationships between ciliate distribution and abundance and their potential food sources along the salinity gradient of an eutrophic estuary.It is hypothesised that despite the strong longitudinal changes in salinity within the estuary,other factors such as availability of bacteria and heterotrophic nanoflagellates(HNF),and the size classes distribution of the phytoplankton must also playa major role in the structure of ciliate assemblages.2.Material and methodsThe study was carried out at the Nervio n River estuary,a small estuary(17km long and20–150m wide) draining into the Cantabric Sea in the Bay of Biscay (43 209N,3 009W)(Fig.1).It is entirely channelled, ending in a semi-enclosed embayment,and receives con-siderable amounts of sewage from a waste treatment plant situated in the middle estuary.Surface water samples were collected monthly from March2000to March2002at8stations(Stations1–8)located along the longitudinal axis of the estuary.At each sam-pling site salinity,temperature and dissolvedoxygen Fig.1.Study area in the Cantabrian Sea(Bay of Biscay).Location of sampling stations and WTP.1170I.Urrutxurtu et al./Estuarine,Coastal and Shelf Science57(2003)1169–1182concentration were measured with a WTW LF197salino-meter and a YSI58(5739)oxymeter.For phytoplankton,HNF and ciliate enumeration, samples were preserved in0.1%glutaraldehyde(final concentration)and stored in the dark at4 C.Samples were examined within2weeks of collection,by settling 10–50ml subsamples(depending on the seston content of the water),following the Utermo hl method.For phytoplankton counts,several transects were analysed at400Âmagnifications counting at least100cells of the most abundant taxa.For ciliates,the whole chamber area was examined at100Âor400Âmagnification with a Nikon Diaphot TMD inverted microscope,equipped with phase contrast and bright-field illumination.Live samples were also examined to facilitate species identi-fication as well as to observe ciliate trophic behaviour. Aloricate ciliates were determined to species level,when possible,following Foissner and Berger(1996).Tin-tinnids were identified following Marshall(1969).In the water column,ciliates can be free swimmers or be attached to particles,mainly to organic macroaggregates of detritus,bacteria and HNF,which in this microen-vironment attain higher sizes than their respective free forms(Artolozaga et al.,2000).In this study,all ciliates encountered in the water samples have been considered as pelagic,despite some of them having been reported as benthic in the literature.Autotrophic and HNF as well as cyanobacteria were observed and enumerated at1000Âmagnification from DAPI-stained samples(Porter&Feig,1980)using a Leica DMRB epifluorescence microscope.Additional water samples(250–1000ml)werefiltered through Whatman GF/Ffilters and kept refrigerated in the dark to quantify chlorophyll a(Chl a)concentration fol-lowing Parsons,Maita,and Lalli(1989).After being extracted in90%acetone for24h,pigments were measured using a Shimadzu UV-160A Spectrophoto-meter.Chl a concentration was estimated by means of the equations of Jeffrey and Humphrey(1975).According to Leakey,Burkill,and Sleigh(1994), samplesfixed with glutaraldehyde(1%final concentra-tion)lost31%of the total ciliate community and45% of the aloricate ciliates compared with samples pre-served in0.4%final concentration of acid Lugol’s iodine paring with5%Bouin’s solution Leakey et al.(1994)observed losses of about16%for the total community and23%for aloricate ciliates.To know the effect of the differentfixatives on the abundance of ciliate population,in this study the samples taken from May2000to September2000at all stations werefixed in parallel with both glutaraldehyde and5%final concen-tration of Bouin’s solution.On the other hand,between March2001and March2002samples were also pre-served in glutaraldehyde as well as in2%acid Lugol’s iodine solution.No significant differences were observed in ciliate abundance betweenfixatives(ANOVA,p>0:05)which agrees with Pitta,Giannakourou,and Christaki(2001)who obtained similar results when com-paring water samplesfixed with both formaldehyde and acid Lugol’s iodine solution.Statistical analyses were performed using SPSS7.5.1 statistical package.Cell concentrations were logðxþ1Þtransformed prior to the principal component analysis (PCA).3.Results3.1.Environmental conditionsSurface water temperature exhibited the typical sea-sonal pattern of warm temperate waters,with maximum values up to22 C in summer and minimum of9 C in winter.Temperature showed higherfluctuations in the inner than in the outer estuary.Surface salinity,measured using the Practical Salinity Scale,ranged from0.2to34.8depending on the river flow conditions,and reflected well the residence time of the water,much longer in summer.After heavy rains (November2000,April2001and February2002)surface salinity decreased drastically along the entire estuary, but especially in the inner zone where salinity dropped to less than0.5.Generally,a polyhaline water mass (>30)of marine origin,penetrated in the estuary through the river bed,creating a marked halocline in the inner estuary.Chl a concentration varied from0.11l g lÿ1in Decem-ber2001to22.55l g lÿ1in September2001.The higher concentrations were observed during late spring–sum-mer in the inner estuary,coinciding with water tempera-tures above18 C.Dissolved oxygen concentration remained relatively high from November to May,decreasing drastically in June,mainly in the middle estuary,where it remained at low levels during all the summer.Values below 1.5mg lÿ1were registered in this zone during summer, although this seasonal decrease was less marked the second year.3.2.Total ciliate abundance and distributionCiliate abundance ranged from100cells lÿ1in Octo-ber2000at Station7to5.4Â105cells lÿ1in June2000at Station4.In this study,we observed noticeable differ-ences in ciliate abundance between the two seasonal cycles.During both years,the highest densities(above 105cells lÿ1)were registered in late spring–summer, although thefirst year ciliate abundance was more than two-fold higher and more evenly distributed along the estuary than the second year.Ciliate abundancefluctuated markedly on a seasonal as well as on a spatial scale.Cell concentrations were1171I.Urrutxurtu et al./Estuarine,Coastal and Shelf Science57(2003)1169–1182relatively low from autumn to early spring,blooming in late spring and maintaining or increasing their density in summer.The highest concentrations appeared at Station 4,in the middle estuary,and decreased to the inner and outer parts of the estuary.3.3.Ciliate community structureDuring the study period,a total of135taxa were identified,most of them to species level,and assigned to 20orders according to Small and Lynn(1985):Hetero-trichida,Armophorida,Choreotrichida,Oligotrichida, Stichotrichida,Prostomatida,Prorodontida,Haptorida, Pleurostomatida,Pharyngophorida,Cyrtophorida,Exo-genida,Endogenida,Evaginogenida,Microthoracida, Peniculida,Euplotida,Hymenostomatida,Scuticocilia-tida,Sessilida and Plagiopylida(Table1).The order Choreotrichida was further divided into two subgroups: tintinnids and naked choreotrichs.Scuticociliates,oligotrichs and naked choreotrichs dominated the community in terms of number.These groups were present all around the estuary and through-out the sampling period,contributing about95%to total ciliate abundance.Fig.2shows the average ciliate abundance and the percentage contribution of the most abundant taxa along the estuary.In the outer estuary(Stations1and2), the polyhaline conditions(>30)favoured the presenceTable1List of ciliate taxa found in the Nervio n River estuary from March2000to March2002Ciliate taxaAcineria incurvata Holosticha pullaster Stichotricha aculetaAcineta tuberosa Laboea strobila Strombidinopsis acuminatum Amphileptus procerus Lacrymaria coronata Strombidinopsis elongata Askenasia volvox Leegaardiella sp.Strombidinopsis minima Aspidisca cicada Lembadion lucens Strombidium conicum Aspidisca lynceus Litonotus alpestris Strombidium cornucopidae Aspidisca fusca Litonotus cygnus Strombidium virideAspidisca steini Litonotus lamella Strombilidium caudatum Astylozoon fallax Lohmaniella spiralis Strombilidium conicum Balanion sp.Loxophyllum helus Strombilidium elegans Blepharisma sp.Mesodinium pulex Strombilidium minimum Caenomorpha sp.Metacineta sp.Stylonychia mytilus complex Carchesium polypinum Metacylis jo¨rgensenii Stylonychia pustulata Chaetospira sp.Metopus contortus Tachysoma pellionellum Cinetochilum margaricetum Monodinium balbiani Tetrahymena pyriformis complex Cinetochilum marinum Myrionecta rubra Tiarina fususCodonella elongata Opercularia articulata Tintinnopsis balticaColeps hirtus Opercularia coarctata Tintinnopsis beroidea Cothurnia sp.Oxytricha hymenostoma Tintinnopsis campanula Cyclidium glaucoma Paramecium caudatum Tintinnopsis cylindrica Cyrtostrombidium sp.Paramecium putrinum Tintinnopsis levigata Dadayiella ganymedes Paramecium trichium Tintinnopsis parvaDictyocysta elegans Phascolodon vorticella Tokophrya infusionum Didinium gargantua Placus luciae Tontonia appendiculariformis Diophrys appendiculata Plagiopyla sp.Tontonia gracillima Discocephalus minimus Pleuronema coronatum Trachellophyllum apiculatum Drepanomonas sp.Podophrya sp.Trichophrya sp.Dysteria sp.Prodiscophrya sp.Trithigmostoma cucullulus Epistylis chrysemydis Proplectella claparedei Trithigmostoma steiniEpistylis coronata Proplectella parva Urocentrum turboEpistylis hentscheli Prorodon ellipticus Uroleptus lacteusEuplotes spp.Prorodon marinus Uronema nigricansEutintinnus latus Prorodon niveus Uronychia transfugaFavella ehrenbergii Pseudochilodonopsisfluviatilis Urotricha agilisFavella serrata Pseudovorticella monilata Urotricha armataFrontonia acuminata Salpingella decurtata Urotricha globosaFrontonia atra Spathidium sp.Vaginicola ingenitaGlaucoma scintillans Spaherophrya magna Vorticella aquadulcis complex Halteria sp.Spirostomum minus Vorticella campanula Helicostomella subulata Spirostomum teres Vorticella convallaria complex Heliophrya rotunda Steenstrupiella steenstrupii Vorticella infusionum complex Holophrya discolor Stenosemella nivalis Vorticella microstoma complex Holophrya ovum Stenosemella ventricosa Vorticella octavaHolosticha kessleri Stentor niger Vorticella pictaHolosticha monilata Stentor roeselii Zoothamnium procerius1172I.Urrutxurtu et al./Estuarine,Coastal and Shelf Science57(2003)1169–1182of large tintinnids (commonly Tintinnopsis spp.)andhaptorid ciliates (Mesodinium spp.).However,these two groups only contributed between 2.4and 4.5%to total ciliate community in this area,whereas Scuticociliates of the genera Uronema and Cyclidium ,small oligotrichs of the genus Strombilidium and naked choreotrichs of the genus Strombidium were the most abundant taxa.In the middle estuary (Stations 3–5),where the high-est average ciliate abundance was registered,the commu-nity was almost exclusively dominated by scuticociliates,small oligotrichs and naked choreotrichs.Euplotids,although to a much lesser extent in terms of number,were also a noticeable component of the ciliate commu-nity in this area.The lowest ciliate abundance was found in the inner estuary (especially in Stations 7and 8),where Scutico-ciliatida was also the most important group.However,in contrast to the other estuarine segments,oligotrichs and naked choreotrichs decreased in importance,being substituted,among others,by sessilids which appeared in this estuarine segment in noticeable concentrations,comprising up to 22%of the total community at Sta-tion 8.Besides,large species belonging to the orders Hymenostomatida such as Glaucoma scintillans and Tetrahymena pyriformis complex,Peniculida with Para-mecium spp.and Cyrtophorida with Dysteria spp.and Trithigmostoma spp.,were restricted to this area.In Fig.3the percentage abundance of the main ciliate groups as well as the total ciliate abundance have beenrepresented for each sampling station during the two years cycle.Scuticociliates,oligotrichs and naked chor-eotrichs dominated the seaward end (Stations 1,2and 3).A peak of abundance was registered in July 2000due to these three groups with a minor peak in summer 2001.The relative importance of other groups,such as tintinnids,sessilids,haptorids,prorodontids and euplo-tids were only evident during the periods of low cili-ate abundance.The importance of tintinnids decreased from Station 1to 3,the latter being the limit of the distribution of this group within the estuary.Scuticociliates,oligotrichs and naked choreotrichs also dominated in the middle estuary during the peaks of ciliate abundance.Other groups,such as sessilids,euplotids,prorodontids and pleurostomatids increased their relative importance during the minima of ciliate.Peaks of abundance were recorded in summer and the highest ciliate abundance of the whole estuary was mea-sured at Station 4.Ciliate abundance decreased towards the inner estuary (Stations 6,7and 8).Although scuticociliates,oligotrichs and naked choreotrichs were the most abundant taxa,the presence of other groups was more relevant than in the previous stations.3.4.Seasonal dynamics and spatial distribution of selected ciliate groupsFig.4shows the spatial and temporal distribution of the dominant ciliate groups in the estuary.DuringtheFig.2.Percentage spatial contribution of the most important ciliate taxa from March 2000to March 2002,in the Nervion River estuary.1173I.Urrutxurtu et al./Estuarine,Coastal and Shelf Science 57(2003)1169–1182sampling period,20species of Tintinnina were foundin the Nervion River estuary.Tintinnid ciliates showed a seaward distribution,being restricted to the outer part (Stations 1–3),where salinity was generally above 25.Their highest densities were observed in July of both years and in September and October 2000and 2001,respectively (Fig.4A ),the latter with 7.4Â103cells l ÿ1.Despite appearing mainly in summer,some species of the genus Stenosemella such as S.nivalis and S.ventricosa appeared in winter,with temperatures below 13 C.The agglutinated genus Tintinnopsis was the most common and diverse,with T.baltica ,T.beroidea,Fig.3.Percentage seasonal contribution of ciliate taxa and total ciliate abundance (103cells l ÿ1)in each sampling station.Note the different scale of ciliate abundance between stations.Note also the difference in the temporal scale of Station 1,which lacks 6months data.1174I.Urrutxurtu et al./Estuarine,Coastal and Shelf Science 57(2003)1169–1182T.campanula ,T.cylindrica ,T.levigata ,T.parva and Tintinnopsis sp.T.beroidea was the most abundant tintinnid,accounting for up to 75%of the total tintinnid abundance.Hyaline species such as Favella ehrenbergii ,Helicostomella subulata or Eutintinnus latus were only observed in low densities.Oligotrichs and aloricate choreotrichous ciliates were present throughout the sampling period but they bloomed in summer,with densities close to 1.2Â105cells l ÿ1(Fig.4B ).The group was dominated by small sized taxa (<40l m)such as Strombidium sp.,S.conicum and Strombilidium elegans .Large speciessuchFig.3(continued )1175I.Urrutxurtu et al./Estuarine,Coastal and Shelf Science 57(2003)1169–1182as Strombidinopsis acuminatum and Strombidinopsis elongata were commonly observed in the outer estuary,but always in low concentrations.Mixotrophic spe-cies,represented by Laboea strobila ,Strombidium viride ,Tontonia apendiculariformis and Tontonia gracillima were rather scarce.Scuticociliatida was the most abundant group in theNervion River estuary,reaching 4.9Â105cells l ÿ1in June 2001at Station 4.This group was characterised by small sized ciliates like Cyclidium glaucoma and Uronema nigricans ,which were the most abundant species.Scuti-cociliates were present throughout the sampling period along the entire estuary.They showed a strong sea-sonal variability,predominating in the middle estuary (Stations 2–5)and reaching their maxima in summer (Fig.4C ),when water temperature exceeded 18 C.Euplotids only reached relatively high concentrations (3.6Â103cells l ÿ1)in September 2000and August 2001(Fig.4D ),coinciding with elevated water temperature (>20 C)and salinity (>24)in the middle estuary (Stations 4and 5).Euplotes spp.,Aspidisca cicada and Aspidisca lynceus were the dominant taxa.Sessilids were mainly observed in the inner estuary (Fig.4E ),although they extended to the outer part dur-ing the rain pulses of April 2000and November 2001.Vorticella was the most abundant and common genus with V.aquadulcis complex,V.campanula ,V.convallaria complex,V.infusionum complex,V.microstoma com-plex,V.octava and V.picta ,although species of Epistylis and Opercularia such as E.chrysemydis ,E.coronata ,E.hentscheli ,O.articulata and O.coarctata were also present although in lower concentrations.Hymenostomatids,peniculids and cyrtophorids showed a similar distribution pattern.They were res-tricted to the inner part of the estuary (Stations 7and 8),and showed the highest densities in February and December 2001,when water temperatures were 11.5 C and between 6and 6.4 C,respectively (Fig.4F ).Maximum densities of these groups were observed in February 2001with 2.7Â103cells l ÿ1for hymenostoma-tids and 3.8Â103cells l ÿ1for cyrtophorids.Other ciliate groups were not so abundant,their den-sities being normally below 5Â102cells l ÿ1.Among them,haptorids,represented mainly by Askenasia volvox and Mesodinium pulex ,appeared in summer in the outer estuary (Stations 1–3).The autotrophic species Myrionecta rubra (formerly Mesodinium rubrum )was very rare.Prostomatids and prorodontids did not show any clear spatial or temporal trends along the estuary during the sampling period,Balanion sp.and Urotricha spp.being the most common organisms.Pleurostoma-tids with Litonotus spp.and Acineria spp.,stichotrichs with Holosticha spp.,heterotrichs with Stentor spp.and armophorids with Caenomorpha sp.and Metopussp.Fig.4.Seasonal dynamics and spatial distribution of the most important ciliate groups in the Nervion River estuary from March 2000to March 2002.Abundance (103cells l ÿ1)of (A)Tintinnina,(B)Oligotrichida and naked choreotrichida,(C)Scuticociliatida,(D)Euplotida,(E)Sessilida and (F)Hymenostomatida and Cyrtophorida.1176I.Urrutxurtu et al./Estuarine,Coastal and Shelf Science 57(2003)1169–1182as the main representatives,were related to freshwater and restricted to the inner part of the estuary.Among suctorids,the endogenid Acineta tuberosa was the most common species.This group appeared mainly in the middle and inner estuary,always in low concentrations. Drepanomonas sp.was the only microthoracid ciliate present in the Nervio n River estuary.It was restricted to the inner part and appeared in winter.Plagiopylida was the less common ciliate group,with only one taxa (Plagyopila sp.)that appeared only once throughout the sampling period at Station4.3.5.Abundance and distribution of potential food sources for ciliateIn addition to bacteria,ciliates may consume phytoplankton,HNF as well as other ciliates.Among phytoplankton,the following groups have been de-lineated during the two years survey in the Nervio n River estuary:dinoflagellates,cyanobacteria of the genus Synechococcus,diatoms,green algae and autotro-phicflagellates.HNF have also been evaluated.All these groups showed a clear seasonal dynamic,peaking in late spring–summer.Dinoflagellates and Synechococcus were restricted to the outer estuary(Stations1–3),denoting their marine origin.The former reached their maximum densities (3.3Â106cells lÿ1)in late summer(Fig.5A),Prorocen-trum minimum,with2.8Â106cells lÿ1,being the most abundant species.The genus Heterocapsa,with H. Pygmaea,was also abundant.The most abundant dinoflagellates were small sized species.In live samples, the heterotrophic dinoflagellate Oxyrrhis marina was observed in great numbers in the middle estuary.The cyanobacteria of the genus Synechococcus was the most abundant group in terms of number,reaching values up to2Â107cells lÿ1in August2001(Fig.5B).Dinoflagel-lates and cyanobacteria only penetrated up the estuary in September2000and August2001,coinciding with a deep intrusion of marine water.Green algae were mainly from freshwater origin and showed a clear seasonal distribution,reaching the highest cell concentrations also in late spring–summer (Fig.5C).Higher densities,above5Â105cells lÿ1,were recorded in the inner part(Stations6–8).Various Ankistrodermus and Scenedesmus species were the more abundant taxa.Diatoms formed one of the most important groups in the Nervio n River estuary.They were present through-out the entire sampling period being widely distributed along the estuary due to the high concentrations reached by freshwater species,mainly Thalassiosira weissflogii and Cyclotella meneghiniana and marine species,among which Pseudo-nitzschia seriata and Skeletonema costa-tum were the most abundant and recurrent species(Fig. 5D).The latter was the most abundant diatomduring Fig.5.Seasonal and spatial distribution of the phytoplankton assemblages in the Nervio n River estuary during the sampling period.Abundance (104cells lÿ1)of(A)dinoflagellates,(B)Synechococcus sp.,(C)diatoms,(D)green algae,(E)autotrophicflagellates and(F)heterotrophicflagellates.1177I.Urrutxurtu et al./Estuarine,Coastal and Shelf Science57(2003)1169–1182the sampling period,reaching values above4Â106 cells lÿ1in July and August2001.Also widely distributed along the estuary were small flagellates,both autotrophic and heterotrophic forms. Autotrophic nanoflagellates(ANF)reached the highest densities in summer,and were more abundant in the middle and outer estuary(Fig.5E),with concentrations above3Â106cells lÿ1.HNF were more abundant than autotrophic forms and,in contrast with these,their highest concentrations were recorded in the inner and middle estuary(Fig.5F). Maximum cell concentrations of this group were close to 107cells lÿ1.Dominantflagellates were the prasinophyte Nephroselmis minuta and the cryptophytes Teleaulax acuta and Hemiselmis sp.The raphidophyte Hetero-sigma akashiwo was found in the middle estuary in bloom proportions in June2000.This species was ob-served in other occasions although in lower numbers.4.Discussion4.1.The study areaPeculiar features of the Nervio n River estuary,which might affect the pelagic communities directly as well as indirectly throughout their affect on benthic communi-ties,are that it lacks intertidal shallows,being entirely channelled,and that it receives the effluent of a domestic WTP,which drains in the middle estuary,near Station4.As a consequence,the estuary is eutrophic and ex-periences episodic events of hypoxia or even anoxia in summer(Uriarte,personal communication),resulting in an impoverished benthic invertebrate fauna(Gonza lez-Oreja,personal communication).This author observed that the macrozoobenthos,which was dominated by Oligochaeta,reached relatively high concentrations only in the outer estuary,with densities up to1.1Â104indi-viduals mÿ2.In the middle and inner estuary macro-zoobenthos density ranged between total absence and 80individuals mÿ2.In these conditions,it is presumed that the pelagic webs are the main trophic pathways in the estuary and remain unchecked by benthicfilter-feeders for most of its length.4.2.Ciliate community structure and distributionScuticociliates,oligotrichs and choreotrichs were the dominant taxa in the estuary and were characterised by small sized taxa(<40l m)all along the rger taxa were more frequent in the outer estuary but in low numbers.The dominance of scuticociliates,oligotrichs and choreotrichs seems to be a common feature in estuaries and coastal waters(Dolan,1991;Laybourn-Parry,Rogerson,&Crawford,1992;Muylaert,Van Mieghem,Sabbe,Tackx,&Vyverman,2000;Sherr& Sherr,1987)as well as in freshwater systems(Hadas and Berman,1998).Small aloricate ciliates<20l m were also dominant in a nearby coastal area of the southern Bay of Biscay,contributing about63%to total ciliate abundance(Quevedo&Anado n,2000).The size of the ciliate assemblages has been related to the trophic status of the water,with small forms,mainly bacterivorous, dominating in eutrophic conditions whereas large bodied forms,mainly phytophagous,are characteristic of oligotrophic waters(Beaver and Crisman,1982).The dominance of small sized ciliates denotes the eutrophic condition of the Nervio n River estuary,especially in the middle part.During the survey,20species of tintinnids were identified in the outer estuary.In agreement with the typical estuarine pattern,tintinnids were more abundant at the seaward,although Dolan&Gallegos(2001) found,in contrast with this trend,a maximum of tintinnids abundance and diversity in the mesohaline (14–17)part of the Chesapeake Bay.Tintinnid species richness was comparable to that found in other coastal waters(Dolan et al.,2001;Kamiyama&Tsujino,1996).Meanwhile,tintinnid abundance in the Nervio n River estuary compares well with other data reported for estuarine and coastal waters(Kamiyama&Tsujino, 1996;Pierce&Turner,1994).The latter authors ob-served that the abundance of many tintinnids were as-sociated with temperature,the<20l m size fraction of chl a and the water column stability.Pierce and Turner (1994)also found that temperature seems to exert a major influence on species succession around the year although temperature does not appear to directly cor-relate with total tintinnids abundance.In the present study,tintinnids appeared in the estuary during the warmer period,although a direct relationship with temperature cannot be established from this parallelism.The ciliate concentrations registered in the Nervio n River estuary are among the highest reported in the literature for other estuaries and coastal waters(Dolan &Coats,1990;Pierce&Turner,1992;Sime-Ngando, Gosselin,Roy,&Chanut,1995;Vincent&Hartmann, Table2Ciliate population densities(cells lÿ1)at different locationsLocationCiliate density(cells lÿ1)ReferenceFlorida(hypereutrophicpond)Max.214000Beaver and Crisman(1982)Limfjorden1000–160000Andersen and Sorensen(1986)Chesapeake Bay3000–23000Dolan and Coats(1990) Priest pot3700–52000Berninger,Wickham,and Finlay(1993)Lake Kinneret3000–47000Hadas and Berman(1998) Furuike pond22000–3500000Nakano et al.(1998) Schelde estuary Max.450000Muylaert et al.(2000) Nervio n Riverestuary100–537000This study1178I.Urrutxurtu et al./Estuarine,Coastal and Shelf Science57(2003)1169–1182。
Spatial and Seasonal Variations of Heavy Metals in Wetland Soils

(Received December 20, 2012; revised May 25, 2013)
ABSTRACT
The environment of estuarine wetlands has been attracting worldwide attention. To study the spatial distribution of pollutants in the tidal flats of the Yangtze Estuary, Southeast China, the Eastern Tidal Flat of Chongming Island (EC) and the Jiuduansha Shoal (JS) of the estuary were selected as the study sites. At each of the two sites, a cross-transect from land to sea was established and topsoil and soil core samples in the cross-transect were collected spatially and seasonally to determine their contents of heavy metals (Cu, Zn, Pb, Cd, Cr, Ni, Mn, and Fe) and grain-size characteristics. The results showed that the heavy metal loads were commonly higher in the soils of nearshore high tidal flats and had a tendency of decreasing from land to sea at both of the study sites. The contents of heavy metals in the soils of the high and medial tidal flats were mostly higher in April and November but lower in July. Corresponding spatial and seasonal variations in grain size of the intertidal soils were also observed at the two study sites. The soils in the nearshore high tidal flats were finer and gradually got coarser seawards; they were relatively finer in April and November but coarser in July. Furthermore, the contents of heavy metals in the intertidal soils of both the sites EC and JS were significantly positively correlated with the clay (< 2 μm) and 2–20 μm fractions, but negatively with the sand (> 63 μm) and 20–63 μm fractions, which suggested that the heavy metals in the intertidal soils were primarily combined with the fine particulate fraction ( < 20 μm), especially clay, and hence the spatial and seasonal variations in heavy metals were actually caused by the change of the grain-size characteristics of the intertidal soils due to the different sedimentary environments in the estuary. The results of this study may also contribute to a better understanding of the soil formation and classification in the tidal flats of the Yangtze Estuary. Key Words: clay fraction, grain-size characteristics, intertidal soils, pollutants, sedimentary environments
外文翻译最后文献段
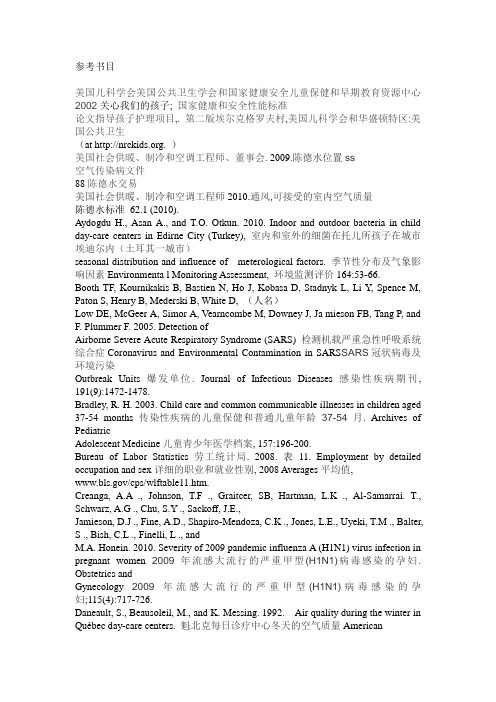
参考书目美国儿科学会美国公共卫生学会和国家健康安全儿童保健和早期教育资源中心2002关心我们的孩子;国家健康和安全性能标准论文指导孩子护理项目,.第二版埃尔克格罗夫村,美国儿科学会和华盛顿特区:美国公共卫生(at . )美国社会供暖、制冷和空调工程师、董事会. 2009.陈德水位置ss空气传染病文件88陈德水交易美国社会供暖、制冷和空调工程师2010.通风,可接受的室内空气质量陈德水标准62.1 (2010).Aydogdu H., Asan A., and T.O. Otkun. 2010. Indoor and outdoor bacteria in child day-care centers in Edirne City (Turkey), 室内和室外的细菌在托儿所孩子在城市埃迪尔内(土耳其一城市)seasonal distribution and influence of meterological factors. 季节性分布及气象影响因素Environmenta l Monitoring Assessment, 环境监测评价164:53-66.Booth TF,Kournikakis B, Bastien N, Ho J, Kobasa D, Stadnyk L, Li Y, Spence M, Paton S, Henry B, Mederski B, White D, (人名)Low DE, McGeer A, Simor A, Vearncombe M, Downey J, Ja mieson FB, Tang P, and F. Plummer F. 2005. Detection ofAirborne Severe Acute Respiratory Syndrome (SARS) 检测机载严重急性呼吸系统综合症Coronavirus and Environmental Contamination in SARS SARS冠状病毒及环境污染Outbreak Units爆发单位. Journal of Infectious Diseases感染性疾病期刊, 191(9):1472-1478.Bradley, R. H. 2003. Child care and common communicable illnesses in children aged 37-54 months传染性疾病的儿童保健和普通儿童年龄37-54月. Archives of PediatricAdolescent Medicine儿童青少年医学档案, 157:196-200.Bureau of Labor Statistics劳工统计局. 2008. 表11. Employment by detailed occupation and sex详细的职业和就业性别, 2008 Averages平均值,/cps/wlftable11.htm.Creanga, A.A ., Johnson, T.F ., Graitcer, SB, Hartman, L.K ., Al-Samarrai. T., Schwarz, A.G ., Chu, S.Y ., Sackoff, J.E.,Jamieson, D.J ., Fine, A.D., Shapiro-Mendoza, C.K ., Jones, L.E., Uyeki, T.M ., Balter, S ., Bish, C.L ., Finelli, L ., andM.A. Honein. 2010. Severity of 2009 pandemic influenza A (H1N1) virus infection in pregnant women2009年流感大流行的严重甲型(H1N1)病毒感染的孕妇. Obstetrics andGynecology2009年流感大流行的严重甲型(H1N1)病毒感染的孕妇;115(4):717-726.Daneault, S., Beausoleil, M., and K. Messing. 1992. Air quality during the winter in Québec day-care centers.魁北克每日诊疗中心冬天的空气质量AmericanJournal of Public Health《美国公共卫生期刊》, 82(3):432-434.De Mello, A.L., Ferreira, E.C., Villas Boas, L.S., and C.S. Panuti. 1996. Cytomegalovirus infection in a day care center in themunicipality of Sao Paulo巨细胞病毒感染在日托中心设在圣保罗. Review of Institu te of Medicine of the Tropics,回顾医学事业有成千上万的热带地区38(3): 165-169.Department of Defense国防部. 2002. Child Development Centers Design儿童发展中心设计. Unified Facilities Crite ria统一设施判据(UFC) 4-740-14, 1 August 2002.Ferng, S.F., Lee, L.W. 2002. Indoor air quality assessment of daycare facilities with carbon dioxide室内空气品质评价日托服务设施和二氧化碳, temperature, and humidity as indicators温度、湿度为指标. Journal of Envi rononmental Healt环境健康日志, 65(4):14-18.Fleming, D.W., Cochi, S.L., Hightower, A.W., and C.V. Broo me. 1987. Childhood Upper Resp iratory Infections小孩上呼吸感染: to whatdegrees is incidence affected by day-care attendance日间托儿发病率程度. Pediatrics 小儿科79:55-60.General Services Administration. 总务管理局2003. PBS-140 Child Care Center Design Guide儿童监护中心的设计指南.Gessner, B.D., Ussery X.T., Parkinson, A.J., and R. F. Breiman. 1995. Risk factors for invasive disease caused bystreptococcus pneumonia among Alaska native children younger th an 2 years of age. Pediatric Infectious Disease Journal阿拉斯加年纪为两岁的小孩受日侵袭性疾病风险因素引起肺炎,儿科感染症医学期刊14: 123-128.Hoti, F., Erastu, P., Leino T., and K. Auranen. 2009. Outbreaks of Streptococcus pneumoniae carriage in day care cohorts inFinland芬兰爆发的肺炎链球菌日托大队马车–Implications for elimination of transmission消除影响的传播. BMC Infectious Diseases, BMC传染病9:102-120. Hurwitz, E.S., Gunn W.J., Pinsky P.F., and L.B. Schonberger. 1991. Risk of respiratory illness associated with day careattendance呼吸相关疾病的风险日托出席: 全国性研究. Pediatrics,小儿科87: 62-69.Jhung, M.A., Swerdlow, D., Olsen, S.J., Jernigan, D., Biggers taff, M., Kamimoto, L, Kniss, K., Reed, C., Fry, A., Brammer,L., Gindler, J., Gregg, W.J., Bresse, J., and Lyn Finelli. 2010. Epidemiology of 2009 Pandemic Influenza A (H1N1) inthe United States.2009年美国流感大流行的流行病学甲型(H1N1)Clinical Infectious Diseas临床感染es. 2011:52 (Suppl 1): S13-S26.Kamper-Jorgensen, M., Wohlfart, J., Simonsen, J., Gronbaek, M., and C.S. Benn. 2006. Population-based study of the impactof childcare attendance on hospitalizations for acute re spiratory infections人群研究的影响住院儿童急性反应呼吸感染. Pediatrics小儿科, 118: 1439-1446. Laughlin, L. 2010. Who’s Minding the Kids?究竟是谁在负责看孩子吗ChildCare Arrangements Spring 2005 and Summer 2006子女托管服务2005年春季和夏季2006. Current PopulationReports现行人口报告P70-121. U.S. Census Bureau人口统计局, Washington DC, 2005.McCluskey, R., Sandin, R. and J. Greene. 1996. Detection of airborne cytomegalovirus in hospital rooms ofimmunocompromised patients检测机载巨细胞病毒免疫缺陷病人的病房. Journal of Virological Methods病毒学方法期刊, 56(1):115-118McDonald D. 1990. Architecture for Kids建筑为孩子: Deinstitutionalizing the design of child care centers儿童看护中心的设计. Day and Early Education早期教育,Summer: 4-8.Mohle-Boetani, J.C., Matkin, C., Pallansch, M., Helfand, R., Fenstersheib, M., Blanding, J.A., and S.L. Solomon. 1999. Viralmeningitis in child care center staff and parents病毒性髓膜炎儿童护理中心的工作人员和父母: an outbreak of echovirus 30 infections艾柯病毒爆发的传染病. Public HealthReports,公共健康报告114(3):249-256.Murph, J.R., Baron J.C., Brown C.K, Ebelhack, CL, and J.F. Bale Jr. 1991. The occupational risk of cytomegalovirusinfection among day care providers职业风险在日间护理提供者巨细胞病毒感染, Journal of the American Medical Association,美国医学协会期刊》上265(5): 603-608.Papineni, R.S., and F.S. Rosenthal. 1997. The size distribution of droplets in the exhaled br eath of health human subjects.颗粒的粒度分布的呼出品牌的健康人体之后Journal of Aerosol Medicine,喷雾医疗期刊10: 105-116.Raynor B.S. 1993. Cytomegalovirus infection in pregnancy孕期巨细胞病毒感染. Seminars in Peri natololgy,环境研讨会17(6):394-402.© 2011 ASHRAE 89Reves R.R., and L.K. Pickering. 1992. Impact of child care on infectious diseases in adults照顾孩子的影响传染病的成年人. Infectious Disease Clinics ofNorth America北美传染病诊所, 6 (1): 239-250.Stelma, F.F., Smismans, A., Goossens V.J., Bruggeman, C.A., and C.J. Hoebe C. J .2009. Occupational risk of humancytomegalovirus and Parvovirus B19人类巨细胞病毒的职业性危险和微小病毒B19, infection in female day care personnel in the Netherlands荷兰日间护理人员感染女性; a study based onseroprevalence.基于血清阳性率的研究European Journal of Clinical Microbiology 临床微生物学欧洲期刊, 28: 393-397.Stevenson, E., Barrios, L., Cordell, R., Delozier, D., Gorman S, Koenig LJ, et al. 2009. Pandemic Influenz a Planning流行病感应规划: Assessingthe Needs of Children评估需要的孩子. American Journal of Public Health美国公共卫生期刊,9 9(附录2): S255-261.Takala, A. K., Jero J., Kela E.k., Ronnberg, P.R., Koskenniemi, E., and J. Eskola. 1995. Risk factors for primary invasivepneumococcal disease among children in Finland芬兰危险因素,儿童原发性浸润性肺炎链球菌疾病. JAMA, 273: 859-864.Tellier. R., 2009. Aerosol transmission of influenza A virus气溶胶传播流感的病毒: a review of new studies. Journal of the Royal Society Interface.新研究的回顾。
英语作文澳大利亚天气变化
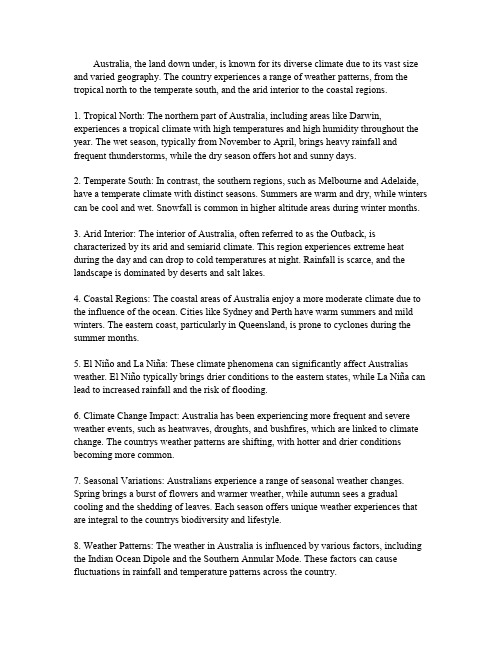
Australia, the land down under, is known for its diverse climate due to its vast size and varied geography. The country experiences a range of weather patterns, from the tropical north to the temperate south, and the arid interior to the coastal regions.1. Tropical North: The northern part of Australia, including areas like Darwin, experiences a tropical climate with high temperatures and high humidity throughout the year. The wet season, typically from November to April, brings heavy rainfall and frequent thunderstorms, while the dry season offers hot and sunny days.2. Temperate South: In contrast, the southern regions, such as Melbourne and Adelaide, have a temperate climate with distinct seasons. Summers are warm and dry, while winters can be cool and wet. Snowfall is common in higher altitude areas during winter months.3. Arid Interior: The interior of Australia, often referred to as the Outback, is characterized by its arid and semiarid climate. This region experiences extreme heat during the day and can drop to cold temperatures at night. Rainfall is scarce, and the landscape is dominated by deserts and salt lakes.4. Coastal Regions: The coastal areas of Australia enjoy a more moderate climate due to the influence of the ocean. Cities like Sydney and Perth have warm summers and mild winters. The eastern coast, particularly in Queensland, is prone to cyclones during the summer months.5. El Niño and La Niña: These climate phenomena can significantly affect Australias weather. El Niño typically brings drier conditions to the eastern states, while La Niña can lead to increased rainfall and the risk of flooding.6. Climate Change Impact: Australia has been experiencing more frequent and severe weather events, such as heatwaves, droughts, and bushfires, which are linked to climate change. The countrys weather patterns are shifting, with hotter and drier conditions becoming more common.7. Seasonal Variations: Australians experience a range of seasonal weather changes. Spring brings a burst of flowers and warmer weather, while autumn sees a gradual cooling and the shedding of leaves. Each season offers unique weather experiences that are integral to the countrys biodiversity and lifestyle.8. Weather Patterns: The weather in Australia is influenced by various factors, including the Indian Ocean Dipole and the Southern Annular Mode. These factors can cause fluctuations in rainfall and temperature patterns across the country.9. Adapting to Weather Changes: Australians have developed various strategies to cope with the countrys diverse weather conditions, from water conservation measures during droughts to bushfire preparedness plans.10. Weather Forecasting: Accurate weather forecasting is crucial for Australians to plan their daily activities, agricultural practices, and to prepare for extreme weather events. The Bureau of Meteorology provides comprehensive weather services and warnings. Australias weather is as diverse as its landscapes, offering a rich tapestry of climatic experiences that shape the countrys environment, economy, and culture. Understanding these weather patterns is essential for both residents and visitors to fully appreciate and navigate the Australian climate.。
Seasonal variations of sediment discharge from the Yangtze River
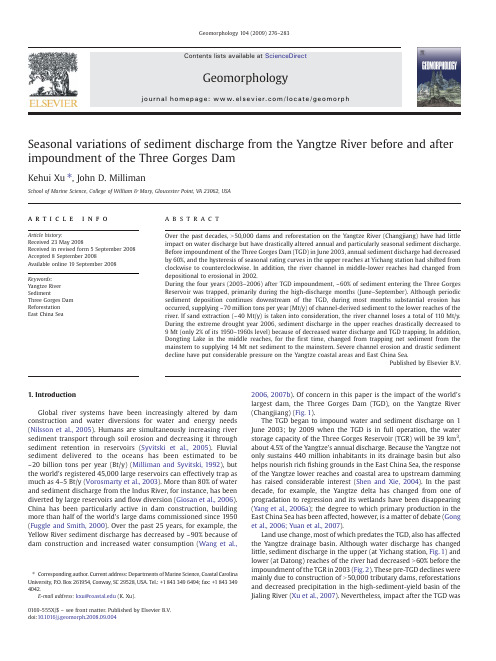
Seasonal variations of sediment discharge from the Yangtze River before and after impoundment of the Three Gorges DamKehui Xu ⁎,John limanSchool of Marine Science,College of William &Mary,Gloucester Point,VA 23062,USAa b s t r a c ta r t i c l e i n f o Article history:Received 23May 2008Received in revised form 5September 2008Accepted 8September 2008Available online 19September 2008Keywords:Yangtze River SedimentThree Gorges Dam Reforestation East China SeaOver the past decades,N 50,000dams and reforestation on the Yangtze River (Changjiang)have had little impact on water discharge but have drastically altered annual and particularly seasonal sediment discharge.Before impoundment of the Three Gorges Dam (TGD)in June 2003,annual sediment discharge had decreased by 60%,and the hysteresis of seasonal rating curves in the upper reaches at Yichang station had shifted from clockwise to counterclockwise.In addition,the river channel in middle-lower reaches had changed from depositional to erosional in 2002.During the four years (2003–2006)after TGD impoundment,~60%of sediment entering the Three Gorges Reservoir was trapped,primarily during the high-discharge months (June –September).Although periodic sediment deposition continues downstream of the TGD,during most months substantial erosion has occurred,supplying ~70million tons per year (Mt/y)of channel-derived sediment to the lower reaches of the river.If sand extraction (~40Mt/y)is taken into consideration,the river channel loses a total of 110Mt/y.During the extreme drought year 2006,sediment discharge in the upper reaches drastically decreased to 9Mt (only 2%of its 1950–1960s level)because of decreased water discharge and TGD trapping.In addition,Dongting Lake in the middle reaches,for the first time,changed from trapping net sediment from the mainstem to supplying 14Mt net sediment to the mainstem.Severe channel erosion and drastic sediment decline have put considerable pressure on the Yangtze coastal areas and East China Sea.Published by Elsevier B.V.1.IntroductionGlobal river systems have been increasingly altered by dam construction and water diversions for water and energy needs (Nilsson et al.,2005).Humans are simultaneously increasing river sediment transport through soil erosion and decreasing it through sediment retention in reservoirs (Syvitski et al.,2005).Fluvial sediment delivered to the oceans has been estimated to be ~20billion tons per year (Bt/y)(Milliman and Syvitski,1992),but the world's registered 45,000large reservoirs can effectively trap as much as 4–5Bt/y (Vorosmarty et al.,2003).More than 80%of water and sediment discharge from the Indus River,for instance,has been diverted by large reservoirs and flow diversion (Giosan et al.,2006).China has been particularly active in dam construction,building more than half of the world's large dams commissioned since 1950(Fuggle and Smith,2000).Over the past 25years,for example,the Yellow River sediment discharge has decreased by ~90%because of dam construction and increased water consumption (Wang et al.,2006,2007b ).Of concern in this paper is the impact of the world's largest dam,the Three Gorges Dam (TGD),on the Yangtze River (Changjiang)(Fig.1).The TGD began to impound water and sediment discharge on 1June 2003;by 2009when the TGD is in full operation,the water storage capacity of the Three Gorges Reservoir (TGR)will be 39km 3,about 4.5%of the Yangtze's annual discharge.Because the Yangtze not only sustains 440million inhabitants in its drainage basin but also helps nourish rich fishing grounds in the East China Sea,the response of the Yangtze lower reaches and coastal area to upstream damming has raised considerable interest (Shen and Xie,2004).In the past decade,for example,the Yangtze delta has changed from one of progradation to regression and its wetlands have been disappearing (Yang et al.,2006a );the degree to which primary production in the East China Sea has been affected,however,is a matter of debate (Gong et al.,2006;Yuan et al.,2007).Land use change,most of which predates the TGD,also has affected the Yangtze drainage basin.Although water discharge has changed little,sediment discharge in the upper (at Yichang station,Fig.1)and lower (at Datong)reaches of the river had decreased N 60%before the impoundment of the TGR in 2003(Fig.2).These pre-TGD declines were mainly due to construction of N 50,000tributary dams,reforestations and decreased precipitation in the high-sediment-yield basin of the Jialing River (Xu et al.,2007).Nevertheless,impact after the TGD wasGeomorphology 104(2009)276–283⁎Corresponding author.Current address:Departments of Marine Science,Coastal Carolina University,P.O.Box 261954,Conway,SC 29528,USA.Tel.:+18433496494;fax:+18433494042.E-mail address:kxu@ (K.Xu).0169-555X/$–see front matter.Published by Elsevier B.V.doi:10.1016/j.geomorph.2008.09.004Contents lists available at ScienceDirectGeomorphologyj o u r n a l h om e p a g e :w w w.e l s e v i e r.c o m /l oc a t e /g e o m o r p himmediate:during the two years after impoundment (2003–2004),sediment discharge at Yichang and Datong decreased by 164and 128million tons (Mt)(Fig.2),respectively,and intense riverbed scouring led to active channel erosion (Xu et al.,2006).This declining trend in sediment transport was further exacerbated by the extreme drought in 2006when flood season water level was lowest in the past 50years (Dai et al.,2008);water discharge decreased by ~30%,and sediment drastically decreased to 9Mt (only 2%of its 1950–1960s level)in the upper reaches at Yichang (Fig.2).During the past decade,numerous studies have been documented water and sediment discharge of the Yangtze River.Long-term sediment flux from the river to the sea since the 1860s was reconstructed using water discharge data collected from the middle reaches (Wang et al.,2008).Statistical analyses of sediment and runoff changes at four mainstem and three tributary stations during the past 55years were done by Zhang et al.(2006).Sediment flux sensitivity toclimate change (e.g.,temperature and precipitation)in upper reaches was documented by Zhu et al.(2008).Flood records in a sediment core collected in the middle reaches and its sediment sources were investigated by Yi et al.(2006).Dam impacts on sediment discharge,channel variations,and delta erosion have been further studied by Yang et al.(2006a,2007)and Yang et al.(2006b).Temporal and spatial variations of basinwide and subbasin discharge of the river were analyzed by incorporating climatic and anthropogenic impacts (Xu et al.,2007).In addition,sediment budget analysis and sand extractions were discussed by Chen et al.(2006,2008),Walling (2007),and Wang et al.(2007a).Although many aspects of water and sediment discharge of the Yangtze have been addressed,few have delineated (i)seasonal rating-curve changes before and after the TGR impoundment,(ii)monthly sediment trapping in the TGR,and (iii)seasonal variations of channel erosion/deposition.These rating-curve changes may signi ficantly impact the timing and pattern of hydrological cycle and sediment transport in the Yangtze basin.Reservoir trapping and channel erosion also play key roles in determining how much sediment can be delivered to the Yangtze estuary to maintain the geomorphology of the Yangtze delta.In addition,quantifying seasonal water and sediment variations is critical for better management of watershed water/sediment resources and to ensure a sustainable development for the Yangtze basin.Our two recent publications (Xu et al.,2006,2007)mainly focused on annual variations using data up to 2004or 2005,respectively,but here we report on the most recent (1950to 2006)seasonal water and suspended-sediment discharge measured at both mainstem and tributary stations.Because the Yangtze usually is divided into upper (above Yichang),middle (Yichang to Hankou)and lower (downstream of Hankou)reaches (Chen et al.,2001),we emphasize both upper (Yichang,directly downstream of the TGD)and lower (Datong,the seaward-most station)reaches.In contrast to other studies,here we (i)show dramatic hysteresis shifting of rating curves,from clockwise to counterclockwise upstream before and after the TGD,(ii)analyze annual and particularly monthly sediment budgets for channels and lakes in the middle-lower reaches,and (iii)attempt to quantify the forcings that caused the drastic Yichang sediment decline to 9Mt in 2006.Because bedload only represents 5%of total sediment discharge (Chen et al.,2001),it is not addressed in our paper except when considering sand extraction from the river channel.Most water and suspended-sediment discharge data are from the Bulletin of Yangtze Sediment (2000,2001,2002,2003,2004,2005,2006,available in /)or Chang-jiang Water ResourcesCommission.Fig.1.The Yangtze River drainage basin.Two grey areas in the southeastern basin are Dongting and Poyang Lakes.Note that Yangtze mainstem delivers water and sediment into Dongting Lake and flows back to the mainstem (shown in arrows).TGD,Three GorgesDam.Fig.2.Temporal variations of annual water and sediment discharge in upper (Yichang station)and lower (Datong station)reaches of the Yangtze River in 1950–2006.Bold vertical dashed lines labeled TGD (Three Gorges Dam)indicate the impoundment in June 2003.Data are from Changjiang Water Resources Commission.Declined sediment discharge before 2003was mainly caused by tributaries damming and reforestation.277K.Xu,liman /Geomorphology 104(2009)276–2832.Mainstem variations at Yichang and Datong 2.1.Temporal variationUnder the control of summer Asian monsoons,water and sediment discharge from Yangtze upper (Yichang)and lower (Datong)reaches both show seasonal patterns.In 1950–1990,more than 70%of water discharge at Yichang and Datong occurred during summer (May –October),with an average peak in July (Fig.3).Water discharge at two stations has shown little change during the past 56years (Fig.2),with peak discharge averaging 80and 130km 3/month (mo),respectively (Fig.3).In June 2003,about 10km 3of water impoundment in the TGR had relatively small impacts on water discharge at two stations.Interestingly,a decrease in water discharge was obvious at Yichang in August 2003(Fig.3);this decline is probably related to decreased precipitation,as no impoundment took place at this time.Sediment discharge at both stations,however,has decreased dramatically since 1950(Fig.2).Peak monthly sediment discharge at Yichang decreased from 160Mt/mo in 1950–1990to only 7Mt/mo in 2006;Datong peak discharge also declined from 110to 17Mt/mo over the same period (Fig.3).Sediment decrease is more drastic in Yichang than that in Datong (Figs.2and 3),mainly because of the compensation from channel erosion downstream of the TGD (discussed later).The timing of peak sediment discharge at both stations,however,shifted from July before 2000to August or September in 2001,2002,2004,and 2005(July is marked by thin dashed lines in Fig.3for comparison).2.2.Cumulative discharge variationThe plots of cumulative sediment discharge versus water discharge (1995–2006)at Yichang and Datong both show stepwise curves,which were fairly steep in summer but gentle in winter (Fig.4).The curve of Yichang began to bend slightly in 2001–2002and became very flat after the impoundment in June 2003.In contrast,the curve at Datong changed more gradually,showing a much smaller impact by the TGD impound-ment (Fig.4).Because the slope of the curve indicatessedimentFig.3.Monthly water and sediment discharge measured in upper (Yichang)and lower (Datong)reaches from 1950to 2006.Note that 1950–1990and 1991–2000are long-term monthly means,followed by monthly discharge from 2001to 2006.For ease of comparison,thin vertical dashed lines represent July for each year;bold vertical dashed lines labeled TGD (Three Gorges Dam)indicate the impoundment in June2003.Fig.4.Cumulative monthly water and sediment discharge at upper (Yichang)and lower (Datong)reaches of the Yangtze River in 1995–2006.The inset shows gentle and steep curves in winter and summer,respectively.TGD (Three Gorges Dam)impoundment in June 2003is highlighted for the comparisons before and after the impoundment.278K.Xu,liman /Geomorphology 104(2009)276–283concentration,sediment concentrations at Yichang in summer were much higher than in winter,with concentrations decreasing rapidly after June 2003.2.3.Rating-curve variationYangtze water mainly comes from the SE owing to higher precipitation,but Yangtze sediment is from the NW because of more erodible soils and steep mountains (Xu et al.,2007).Each year Asian monsoons move northward from the low-sediment-yield SE (in April and May)to the high-yield NW (June –August)(Shi et al.,1985).In upper reaches at Yichang during 1950–1990,sediment concentration in the rising stage (June)on the rating curve was greater than that in the falling stage (October)presumably because there was more easily-erodible sediment available in June (Fig.5).In contrast,lower-reach variations at Datong re flect the combination of spatial source difference and temporal monsoon moving.In 1950–1990Datong's sediment concentration in the rising stage of water discharge (June,sediment-poor)was lower than that in the falling stage (August –September,sediment-rich)during which turbid sediment was supplied by upper reaches (Fig.5).These temporal and spatial settings led to a unique downstream transition on the hysteresis of rating curves from clock-wise at Yichang to counterclockwise at Datong in 1950–1990(Fig.5).This downstream transition,however,has changed gradually since the 1990s.The curves at Yichang switched to being counter-clockwise in 1992and 1995–1997but remained clockwise in the other years of the 1990s,leading its 1991–2000curve to shift to a semi-counterclockwise hysteresis (Fig.5).It then fell and became counterclockwise in 2001–2002,after which it maintained counter-clockwise and dropped further in 2003–2005and 2006.Of these decreases in sediment concentrations at Yichang,the most dramatic is July of 2001–2002when concentration decreased by 60%compared with 1991–2000;in contrast,sediment concentration in August declined by only 20%over the same periods (Fig.5).Downstream at Datong,all the curves since 1991fell below the 1950–1990line but remained counterclockwise (Fig.5).3.Spatial and temporal variationsVariations at Yichang and Datong actually re flect changes on both mainstem and tributaries in the Yangtze basin.As such,our discussion of changes on water and sediment discharge will focus on the transition from the upper to the lower reaches:upstream,inside and downstream of the TGR,respectively.3.1.Upstream of the TGRIn 2006,the upper reaches of the Yangtze experienced an extreme drought,during which water level in flood season was the lowest in the last 50years,a situation called “no flood in the flood season ”(Dai et al.,2008).Annual water discharge at Qingxichang (upstream of the TGR,Fig.1)also reached its lowest level (278km 3/y)in the past half century,~30%less than its long-term (1956–2005)mean (390km 3/y)(Fig.6).Since the year 1986was the beginning of the Yangtze Upstream Water and Soil Conservation Project and 2003is the first TGR impoundment,the plots of water versus sediment discharge at Qingxichang can be divided into three periods:1956–1985,1986–2002,and 2003–2006.Sediment discharge began declining in 1986,and reached its lowest level in 2006(Fig.6).Based on the rating curve for 2003–2006,decreased water discharge in 2006led to a drop of 110Mt in sediment discharge (dashed lines in Fig.6).3.2.Sediment trapping inside the TGRThe 600-km-long TGR is located in the upper stream of the Yangtze,and sediment trapping inside the reservoir has been substantial since the impoundment in 2003.LocatedimmediatelyFig.5.The rating curves of monthly water discharge versus sediment concentration for 1950–1990,1991–2000,2001–2002,2003–2005,and 2006at Yichang and Datong,respectively.Numbers on the curves represent the sequential months (7for July 477and 8for August)of the years,and arrows show the clockwise or counterclockwise hysteresis loops.The curves at Yichang shifted from clockwise in 1950–1990to counterclockwise after2001.Fig.6.The rating curves of annual water discharge versus sediment discharge at Qingxichang station in 1956–1985,1986–2002,and 2003–2006.2006was an extremely dry year during which decreased water discharge led to a 110-Mt decline in sediment discharge.Discharge of Qingxichang was calculated by adding discharge from a nearby upstream station (Zhutuo)to that of two tributaries (at Beibei and Wulong stations)between Zhutuo and the TGR.279K.Xu,liman /Geomorphology 104(2009)276–283upstream and downstream of the TGR,Qingxichang and Yichang stations have been used to monitor the sediment entering and exiting the reservoir,respectively (Fig.1).The contribution from nearby small rivers to the TGR has been regarded as small,although it may reach as high as ~25Mt/y (Yang et al.,2007).During 2002and before TGD impoundment,sediment discharge passing two stations was similar,indicating little net erosion or deposition along the channel between Qingxichang and Yichang (Fig.7).After impoundment,however,124,102,151,and 93Mt of sediment were trapped in 2003,2004,2005,and 2006,respectively,an average of 118Mt/y (Fig.7).During 2003–2006,sediment retention occurred mainly from June to September,a pattern similar to the sediment discharge at Qingxichang (Fig.7).The more supply from upstream of the TGR,the more trapping of sediment inside the reservoir.Monthly sediment discharge entering the TGR correlated with trapped sediment in the TGR (Fig.8);approximately 50%of the sediment entering the TGR was trapped based on the slop of the line in Fig.8.The highest trapping (60Mt)in July 2005corresponded to the highest sediment discharge (90Mt)passing Qingxichang.Inside the TGR,sediment was mainly accumulated near the thalweg and became thicker nearer to the TGD;maximum accumula-tion thickness (53m)occurred 6km upstream of the TGD (Bulletin of Yangtze Sediment,2005,2006).In August 2003,however,sediment discharge at both Qingxichang and Yichang was unusually lower than in the preceding and following months (Fig.7).Because impoundment in the TGR at that time was not signi ficant,this is likely related to decreased water discharge (Fig.3)and reduced precipitation upstream of the TGR,which propagated downstream to Yichang.3.3.Channel erosion downstream of the TGRAnother dramatic change in response to TGR impoundment has been channel erosion downstream of the TGD,which plays a key rolein sediment transfer in the middle and lower reaches.Along the 1140-km stretch between Yichang and Datong,the Yangtze has lost some sediment (due to siltation and reclamation)through several passages (small arrows in Fig.1)connecting Dongting Lake,but has received considerable sediment from tributaries merging at Poyang Lake and the Han River.Prior to 2000,sediment transport in this section displayed extreme seasonal variations,sediment being deposited during high-discharge months (June –September)but subsequently eroded in low-discharge months (Fig.9);net deposition between Yichang and Datong averaged 48Mt/y (Table 1).Deposition down-stream of Yichang declined quickly to 15Mt in 2001,and then reversed to net erosion (−46Mt/y)in 2002(Table 1),erosionoccurringFig.7.Monthly sediment discharge at Qingxichang (A)and Yichang (B),and trapped sediment in the TGR (C).The tributary upstream of Qingxichang is Jinsha River.Discharge of Qingxichang was calculated by adding discharge from a nearby upstream station (Zhutuo)to that of two tributaries (at Beibei and Wulong stations)between Zhutuo and theTGR.Fig.8.Suspended sediment discharge entering the Three Gorges Reservoir (TGR)in 2003–2006correlates with monthly trapped sediment in the TGR.The gauging stations measuring discharge entering the TGR is Qingxichang.280K.Xu,liman /Geomorphology 104(2009)276–283in 10of 12months (Fig.9).In 2002,for the first time,sediment discharge at Datong exceeded that at Yichang (Table 1).As these changes occurred before TGD impoundment,clearly human activities (e.g.,damming and reforestation)on the Yangtze tributaries had collectively played a key role in this deposition-to-erosion transition.After the 2003impoundment,channel erosion occurred in every month in 2003and 2006and most months in 2004–2005(Fig.4)in response to the release of water from the TGR with sharply reduced sediment concentrations.In 2003–2006,an average of ~70Mt/y of sediment was eroded between Yichang and Datong,mostly re flecting the abrupt summertime change from deposition to erosion (Fig.9).Channel erosion mainly took place 630km directly downstream from the TGD (Yichang to Hankou)but diminished farther downstream from Hankou to Datong (Figs.1and 9;Table 1).Because channel-eroded sediment was generally coarser than suspended sediment,median grain size increased from 5μm at Yichang to 11μm down-stream at Hankou in 2005(Bulletin of Yangtze Sediment,2005).4.DiscussionDuring the past half century,human activities have increased in the Yangtze basin.Since 1986,the Yangtze Upstream Water and Soil Conservation Project has constructed nearly one million ponds,reservoirs,and levees,and has increased vegetation cover by 23%;these land conservation activities alone are believed to be responsible for the trapping of ~60Mt of sediment in the upper reaches (Bulletin of Yangtze Sediment,2003).By 2000,more than 50,000dams had been constructed throughout the Yangtze basin.The DanjiangkouDam in the north,for example,has decreased N 95%of sediment discharge from the Han River (Fig.1)since 1968(Yang et al.,2006b ).4.1.Shifting of rating curvesThese human activities have greatly in fluenced hydrological seasonality and hysteresis of the rating curve in the Yangtze River.Upstream of Yichang,sediment concentration experienced the most dramatic decrease in July of 2001–2002(Fig.5),and peak sediment discharge shifted from July before 2000to August –September in 2001–2002and 2004–2005(Fig.3).The July decline played a major role in the shift of the rating curve from clockwise to counter-clockwise.These drastic sediment drops at Yichang are explained by the effect of numerous reservoirs in tributaries of the river as follows.When precipitation is low in January –May,water levels in most reservoirs fall to meet domestic and agricultural needs.With the re-initiation of monsoonal summer rains in the upper reaches (usually in late June and July),reservoirs begin filling,thereby trapping both sediment and water.Once filled in August –September,water and sediment again are discharged from reservoirs.One result of reservoir trapping has been the signi ficant temporal decrease in median grain size of suspended sediment at Yichang,from 22μm in 1950–2000to only 5μm in 2005(Bulletin of Yangtze Sediment,2005).4.2.Sediment budget and complicationsSediment budget provides a way to understand the distribution of sediment in different parts of a river basin (Wang et al.,2007a).Fig.9.Monthly channel deposition (+)/erosion(−)between Yichang and Datong stations,calculated by subtracting Datong from the sum of Yichang,Dongting Lake,Poyang Lake,and Han River.The net depositional pattern in 1950–2000gradually shifted to erosional in 2002,and more signi ficantly in 2003–2006.Note that monthly data of Dongting,Poyang,and Han before 2002are not available;the pre-2002plot represents the difference between the discharge at Yichang and that at Datong.Annual variations are shown in Table 1.Table 1Sediment budget in the middle-lower reaches of the Yangtze River (unit:Mt/y)Year Yichang Dongting Lake Han River Hankou Poyang Lake Datong Channel deposition(+)/erosion(−)Yichang to Hankou (630km)Hankou to Datong (510km)Yichang to Datong (1140km)1950–2000501−86564041043367−19482001299−23328512276−621152002228−16323914275−24−22−46200398−31416518206−56−23−792004640513614147−673−642005110−81717416216−55−26−8120069143581485−32−13−45Yichang,Hankou and Datong are three mainstem stations from upper to lower streams,and Dongting Lake,Han River and Poyang Lake (all italicized)deliver tributary discharges into the mainstem (Fig.1).The Yangtze generally loses sediment into Dongting Lake and receives sediment from Poyang Lake and Han River.Channel deposition(+)/erosion(−)from Yichang to Datong was calculated by subtracting Datong from the sum of Yichang,Dongting,Poyang and Han.The budgets for Yichang –Hankou (630km channel length)and Hankou –Datong (510km)were calculated in a similar way.All channel budgets are shown in bold.Dongting sediment itself was calculated by subtracting four passages flowing into Dongting Lake from one passage going back to the mainstem (Fig.1;Xu et al.,2007).See Fig.9for monthly variations.281K.Xu,liman /Geomorphology 104(2009)276–283Sediment discharge delivered to the lower reaches at Datong is dependent on(i)sediment discharge at Yichang,(ii)net sedimentflux between Dongting Lake and mainstem,(iii)discharge from un-gauged small rivers,(iv)contributions from the Han River and Poyang Lake,as well as(v)sand extraction along channels in the middle-lower reaches (Fig.1;Chen et al.,2008).Based on our sediment-budget analysis, about70Mt/y of channel-derived sediment was eroded and delivered to the lower reaches in2003–2006(Table1).This budget analysis, however,does not take into account small ungauged rivers in Yichang–Datong and sand extraction.Considering the low-sediment yield in the middle-lower reaches,however,contribution from small ungauged rivers should be fairly small.Sand extraction in the middle and lower Yangtze basin began as early as the1950s.In the1980s,sand extraction rapidly increased to 40Mt/y and reached~80Mt/y in the late1990s(Wang et al.,2007a). In2003the Yangtze River Conservation Commission issued a regulation that total sand extraction/mining should be b34Mt/y,but the ban on illegal sand extractions has become a challenge to Chinese government(Chen et al.,2006).Actual sand extraction in2003–2006 may have between34and~80Mt/y.One complicated issue for the budget analysis here is that extracted sand(N64μm)is much coarser than the suspended sediment(5–11μm in median size).When sands are extracted from river bed or banks,there may be more space for suspendedfine sediment to deposit on the bed.If we assume40Mt/y of sand extraction combined with70Mt/y channel erosion(Table1), the river channel could have lost as much as110Mt/y in2003–2006. The resulting channel erosion could result in river bank landslides and levee failures,endangering theflood control and navigation in the middle-lower reaches.Dongting Lake is the major lake located in the middle Yangtze reaches,receiving discharge from the mainstem and southern tribu-taries,which thenflows back into the mainstem(arrows in Fig.1).Net escape of sediment from mainstem to lake has decreased from86Mt/y in1950–2000to16Mt in2002(Table1).Because of active channel erosion downstream of the TGR after June2003,bed level on the mainstem channel was lowered and sediment escape from the mainstem to lake essentially stopped in2004(0Mt).During the extreme drought year2006,sharply dropped water discharge and TGR trapping decreased by110and93Mt sediment(Figs.6and7)in upper stream,respectively,leading only9Mt sediment passing Yichang. Because of sediment starvation in the mainstem,sediment transport changed from escaping from the mainstem before2005to supplying net sediment(14Mt)back to the mainstem in2006(Table1).The area of Dongting Lake has decreased from4350km2in1949to 2623km2in1995because of extensive reclamation and severe siltation(Bulletin of Yangtze Sediment,2000).Dongting Lake has been the major freshwater source for millions of people in the middle reaches of the Yangtze.How to maximize the longevity of Dongting Lake has long been a serious question.This new lake-to-mainstem transport pattern,however,might alleviate the shrinking of the lake and facilitate the management of freshwater resources in the middle reaches.5.ConclusionsBefore the impoundment of the Three Gorges Reservoir(TGR)in 2003,the seasonality of hydrological processes in the Yangtze upper reaches had been altered by tributary dams and reforestation such that the hysteresis of the rating curve in the upper reaches shifted from clockwise to counterclockwise.After the impoundment,60%of the sediment entering the TGR was trapped inflood seasons. Downstream of the TGR,substantial channel erosion happened most of the time in2003–2006.In the extreme drought year2006, sediment discharge in the upper reaches drastically decreased to only2%of1950–1960s level owing to both reduced water discharge and TGR trapping.As downstream channel erosion(70Mt/y)has not yet counteracted TGR trapping(118Mt/y),sediment delivered to the Yangtze estuary will probably continue to decrease,putting pressure on the Yangtze lower reaches and adjacent coastal areas.AcknowledgementsThis research was supported by the U.S.National Science Foundation(NSF)and Office of Naval Research(ONR).We thank Prof.Z.S.Yang(Ocean University of China)for providing part of the hydrological data used in this study.We are grateful to two anonymous reviewers for their comments,suggestions,and critical reviews.ReferencesBulletin of Yangtze River Sediment,2000.Press of Ministry of Water Resources of the People's Republic of China.website:/.Bulletin of Yangtze River Sediment,2001.Press of Ministry of Water Resources of the People's Republic of China.website:/.Bulletin of Yangtze River Sediment,2002.Press of Ministry of Water Resources of the People's Republic of China.website:/.Bulletin of Yangtze River Sediment,2003.Press of Ministry of Water Resources of the People's Republic of China.website:/.Bulletin of Yangtze River Sediment,2004.Press of Ministry of Water Resources of the People's Republic of China.website:/.Bulletin of Yangtze River Sediment,2005.Press of Ministry of Water Resources of the People's Republic of China.website:/.Bulletin of Yangtze River Sediment,2006.Press of Ministry of Water Resources of the People's Republic of China.website:/.Chen,Z.,Li,J.,Shen,H.,Wang,Z.,2001.Yangtze River of China:historical analysis of discharge variability and sedimentflux.Geomorphology41(2–3),77–91.Chen,X.,Zhou,Q.,Zhang,E.,2006.In-channel sand extraction from the mid-lower Yangtze channels and its management:problems and challenges.Journal of Environmental Planning and Management49(2),309–320.Chen,X.,Yan,Y.,Fu,R.,Dou,X.,Zhang,E.,2008.Sediment transport from the Yangtze River,China,into the sea over the Post-Three Gorge Dam Period:a discussion.Quaternary International186(1),55–64.Dai,Z.,Du,J.,Li,J.,Li,W.,Chen,J.,2008.Runoff characteristics of the Changjiang River during2006:effect of extreme drought and the impounding of the Three Gorges Dam.Geophysical Research Letters35,L07406.doi:10.1029/2008GL033456. Fuggle,R.,Smith,W.,rge Dams in Water and Energy Resource Development in The People's Republic of China(PRC).Country review paper prepared as an input to the World Commission on Dams,Cape Town..Giosan,L.,Constantinescu,S.,Clift,P.D.,Tabrez,A.R.,Danish,M.,Inam,A.,2006.Recent morphodynamics of the Indus delta shore and shelf.Continental Shelf Research26(14),1668–1684.Gong,G.,Chang,J.,Chiang,K.,Hsiung,T.,Hung,C.,Duan,S.,Codispoti,L.A.,2006.Reduction of primary production and changing of nutrient ratio in the East China Sea:effect of the Three Gorges Dam?Geophysical Research Letters33,L07610.doi:10.1029/2006GL025800.Milliman,J.D.,Syvitski,J.P.M.,1992.Geomorphic/tectonic control of sediment discharge to the ocean:the importance of small mountainous rivers.Journal of Geology100(5),525–544.Nilsson, C.,Reidy, C.A.,Dynesius,M.,Revenga, C.,2005.Fragmentation andflow regulation of the world's large river systems.Science308(5720),405–408. Shen,G.,Xie,Z.,2004.Three Gorges Project:chance and challenge.Science304(5671), 681b.Shi,Y.-L.,Yang,W.,Ren,M.-e.,1985.Hydrological characteristics of the Changjiang and its relation to sediment transport to the sea.Continental Shelf Research4(1–2), 5–15.Syvitski,J.P.M.,Vorosmarty,C.J.,Kettner,A.J.,Green,P.,2005.Impact of humans on the flux of terrestrial sediment to the global coastal ocean.Science308(5720),376–380. Vorosmarty,C.J.,Meybeck,M.,Fekete,B.,Sharma,K.,Green,P.,Syvitski,J.P.M.,2003.Anthropogenic sediment retention:major global impact from registered river impoundments.Global and Planetary Change39(1–2),169–190.Walling, D.E.,2007.Global change and the sediment loads of the world's rivers.Proceeding of10th international Symposium on River Sedimentation,Moscow, Russia,August2007,vol.1.Moscow State University,pp.112–130.Wang,H.,Yang,Z.,Saito,Y.,Liu,J.P.,Sun,X.,2006.Interannual and seasonal variation of the Huanghe(Yellow River)water discharge over the past50years:connections to impacts from ENSO events and dams.Global and Planetary Change50(3–4), 212–225.Wang,Z.Y.,Li,Y.,He,Y.,2007a.Sediment budget of the Yangtze River.Water Resource Research43,W04401.doi:10.1029/2006WR005012.Wang,H.,Yang,Z.,Saito,Y.,Liu,J.P.,Sun,X.,Wang,Y.,2007b.Stepwise decreases of the Huanghe(Yellow River)sediment load(1950–2005):impacts of climate change and human activities.Global and Planetary Change57(3–4),331–354.Wang,H.,Yang,Z.,Wang,Y.,Saito,Y.,Liu,J.P.,2008.Reconstruction of sedimentflux from the Changjiang(Yangtze River)to the sea since the1860s.Journal of Hydrology349(3–4),318–332.Xu,K.H.,Milliman,J.D.,Yang,Z.S.,Wang,H.J.,2006.Yangtze sediment decline partly from Three Gorges Dam.EOS Transactions87(19)185,190.282K.Xu,liman/Geomorphology104(2009)276–283。
柯本气候分类法
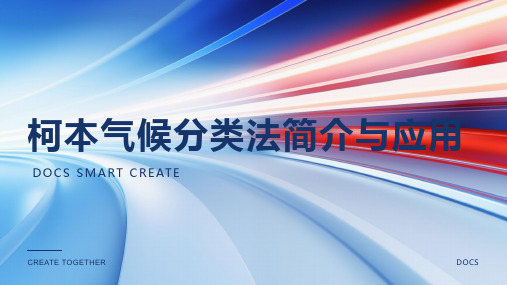
05
柯本气候分类法的优缺点及改进方向
柯本气候分类法的优点与局限性
优点:
• 分类方法简单,易于理解和操作 • 适用范围广,可以应用于全球各地的气候分类 • 分类结果具有较高的实用价值,为气象学、地理学、生态学等领域的研究提供了 依据
局限性:
• 某些气候类型的划分界限较为模糊,容易导致分类结果的不确定性 • 未能充分考虑地形、洋流等地理因素对气候的影响 • 对于极端气候事件的考虑不足,可能导致分类结果的偏差
• C气候类型主要分布在亚洲的东部、东北部,欧洲的东部、北部 等地 • 气候特点:
• 四季分明,气温适中,平均气温在8℃~18℃之间 • 降水分布较均匀,年降水量在500~1000毫米之间 • 夏季温暖多雨,冬季寒冷干燥 • 植被类型:森林、草原等,植物种类丰富,适应性较强
D气候类型:寒带季风气候
柯本气候分类法的分类依据与 指标
• 柯本气候分类法主要依据以下四个指标对气候进行分类 • 平均气温(Mean annual temperature):衡量一个 地区全年气温的平均水平 • 降水量(Precipitation):衡量一个地区全年降水量的多 少 • 干燥度指数(Aridity index):衡量一个地区干燥程度的 指标,计算公式为:降水量/潜在蒸发量 • 季节划分(Seasonal distribution of temperature and precipitation):衡量一个地区气温和降水在不同 季节的分布情况
方面的影响
通过柯本气候分类法,生态学家可以评 估气候变化对生态系统的影响,为生态
保护提供依据
柯本气候分类法在农业、城市规划等领域的应用
农业领域,柯本气候分类法可以用于分析气候对农业生产的影响,如气候对作物生长、病虫害等方面的影响
基于MODIS资料的贵州植被叶面积指数的时空变化及其对气候的响应
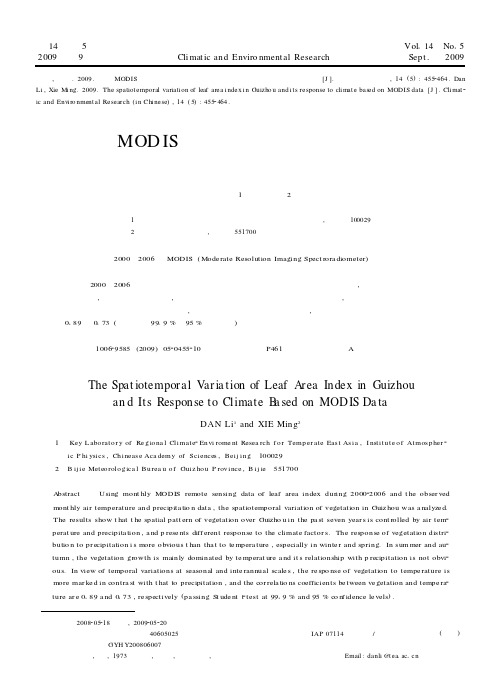
第14卷第5期2009年9月气候与环境研究Cli mat ic and Enviro nment al Research Vol 114 No 15Sept.2009丹利,谢明.2009.基于MODIS 资料的贵州植被叶面积指数的时空变化及其对气候的响应[J ].气候与环境研究,14(5):4552464.DanLi ,Xie Mi ng.2009.The spatiot emporal variati on of leaf area i ndex i n Guizho u and i t s response to cli m at e bas ed on MODIS data [J ].Cli mat 2ic and Envi ro nment al Research (i n C hi nese),14(5):4552464.收稿日期 2008205218收到,2009205220收到修定稿资助项目 国家自然科学基金项目655、中国科学院知识创新工程领域前沿项目I 、财政部科技部公益性行业(气象)科研专项GY Y 6作者简介 丹利,男,3年出生,博士,副研究员,主要从事陆气相互作用与气候模拟研究。
@11基于MOD IS 资料的贵州植被叶面积指数的时空变化及其对气候的响应丹利1 谢明21 中国科学院大气物理研究所东亚区域气候环境重点实验室,北京 1000292 贵州毕节地区气象局,毕节 551700摘 要 利用2000~2006年MODIS (Mode rate Resolution Imaging Spect rora diometer)卫星遥感观测的植被叶面积指数月平均资料以及相应时段的月平均气温、降水的观测资料对贵州植被的时空变化进行了分析。
结果表明贵州2000~2006年植被分布受水热条件控制表现出对气候因子不同的响应关系,其中对降水的响应关系比气温明显,特别是冬季和春季,而植被在夏季和秋季的分布主要受气温的制约,与降水的关系并不明显。
海河流域近17年植被时空变化及其气候影响因素
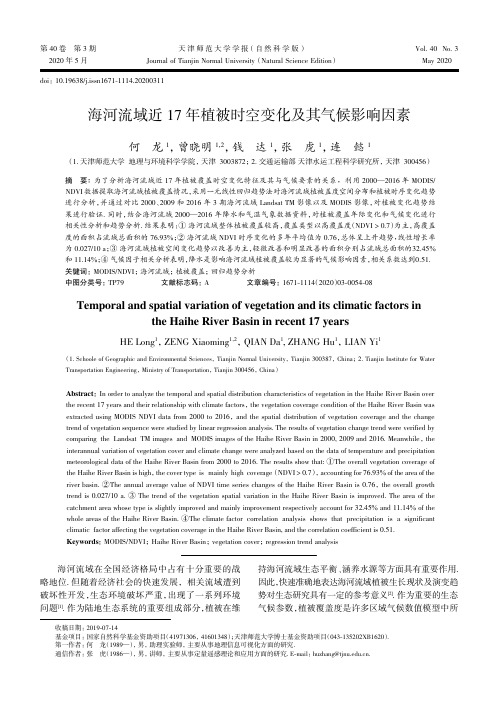
海河流域近17年植被时空变化及其气候影响因素何龙1,曾晓明1,2,钱达1,张虎1,连懿1(1.天津师范大学地理与环境科学学院,天津3003872;2.交通运输部天津水运工程科学研究所,天津300456)摘要:为了分析海河流域近17年植被覆盖时空变化特征及其与气候要素的关系,利用2000—2016年MODIS/NDVI 数据提取海河流域植被覆盖情况,采用一元线性回归趋势法对海河流域植被盖度空间分布和植被时序变化趋势进行分析,并通过对比2000、2009和2016年3期海河流域Landsat TM 影像以及MODIS 影像,对植被变化趋势结果进行验证.同时,结合海河流域2000—2016年降水和气温气象数据资料,对植被覆盖年际变化和气候变化进行相关性分析和趋势分析.结果表明:淤海河流域整体植被覆盖较高,覆盖类型以高覆盖度(NDVI >0.7)为主,高覆盖度的面积占流域总面积的76.93%;于海河流域NDVI 时序变化的多年平均值为0.76,总体呈上升趋势,线性增长率为0.027/10a ;盂海河流域植被空间变化趋势以改善为主,轻微改善和明显改善的面积分别占流域总面积的32.45%和11.14%;榆气候因子相关分析表明,降水是影响海河流域植被覆盖较为显著的气候影响因素,相关系数达到0.51.关键词:MODIS/NDVI ;海河流域;植被覆盖;回归趋势分析中图分类号:TP79文献标志码:A文章编号:1671-1114(2020)03-0054-08Temporal and spatial variation of vegetation and its climatic factors inthe Haihe River Basin in recent 17yearsHE Long 1,ZENG Xiaoming 1,2,QIAN Da 1,ZHANG Hu 1,LIAN Yi 1(1.Schoole of Geographic and Environmental Sciences ,Tianjin Normal University ,Tianjin 300387,China ;2.Tianjin Institute for WaterTransportation Engineering ,Ministry of Transportation ,Tianjin 300456,China )Abstract :In order to analyze the temporal and spatial distribution characteristics of vegetation in the Haihe River Basin overthe recent 17years and their relationship with climate factors ,the vegetation coverage condition of the Haihe River Basin was extracted using MODIS NDVI data from 2000to 2016,and the spatial distribution of vegetation coverage and the change trend of vegetation sequence were studied by linear regression analysis.The results of vegetation change trend were verified by comparing the Landsat TM images and MODIS images of the Haihe River Basin in 2000,2009and 2016.Meanwhile ,the interannual variation of vegetation cover and climate change were analyzed based on the data of temperature and precipitation meteorological data of the Haihe River Basin from 2000to 2016.The results show that:淤The overall vegetation coverage of the Haihe River Basin is high ,the cover type is mainly high coverage (NDVI >0.7),accounting for 76.93%of the area of the river basin.于The annual average value of NDVI time series changes of the Haihe River Basin is 0.76,the overall growth trend is 0.027/10a.盂The trend of the vegetation spatial variation in the Haihe River Basin is improved.The area of the catchment area whose type is slightly improved and mainly improvement respectively account for 32.45%and 11.14%of thewhole areas of the Haihe River Basin.榆The climate factor correlation analysis shows that precipitation is a significantclimatic factor affecting the vegetation coverage in the Haihe River Basin,and the correlation coefficient is 0.51.Keywords :MODIS/NDVI ;Haihe River Basin ;vegetation cover ;regression trend analysisdoi :10.19638/j.issn1671-1114.20200311第40卷第3期2020年5月天津师范大学学报(自然科学版)Journal of Tianjin Normal University (Natural Science Edition )Vol.40No.3May 2020收稿日期:2019-07-14基金项目:国家自然科学基金资助项目(41971306,41601348);天津师范大学博士基金资助项目(043-135202XB1620).第一作者:何龙(1989—),男,助理实验师,主要从事地理信息可视化方面的研究.通信作者:张虎(1986—),男,讲师,主要从事定量遥感理论和应用方面的研究.E -mail :****************.cn.海河流域在全国经济格局中占有十分重要的战略地位.但随着经济社会的快速发展,相关流域遭到破坏性开发,生态环境破坏严重,出现了一系列环境问题[1].作为陆地生态系统的重要组成部分,植被在维持海河流域生态平衡、涵养水源等方面具有重要作用.因此,快速准确地表达海河流域植被生长现状及演变趋势对生态研究具有一定的参考意义[2].作为重要的生态气候参数,植被覆盖度是许多区域气候数值模型中所需的重要信息,也是描述生态系统的重要基础数据[3].归一化植被指数NDVI是利用遥感手段监测地面植物生长状态的一种方法[2],广泛应用于土地覆盖分类[4-6]、植被动态监测[7-9]和植被变化与气候关系[10-13]等诸多研究领域.目前,针对海河流域植被变化已有学者进行了相关研究[14-17],这些研究所采用的NDVI时序数据最高空间分辨率为1km,且所选数据时间序列周期较短,如能加入更长时间序列对植被变化趋势进行分析,结果会更加客观[18].常用的NDVI数据集有GIMMS/NDVI、SPOT VGT/ NDVI和MODIS/NDVI,其中MODIS/NDVI数据具有较高的空间分辨率和数据质量[19-20],可用数据最高空间分辨率为250m,为区域植被趋势研究提供了可使用的时序数据,大大提高了地表植被的观测能力[8].与GIMMS/ NDVI数据相比,MODIS/NDVI数据在可见光波段提高了对叶绿素的敏感度,减少了大气和观测视角等外部因素以及叶冠背景等内在因素的影响,在近红外波段排除了大气水汽的干扰.因此,在植被变化监测中具有较高的准确性[21].本研究利用2000—2016年MODIS/NDVI数据和国家气象信息中心中国地面降水和气温日值数据集,提取海河流域植被覆盖状况,对海河流域近17年植被覆盖变化趋势进行分析,并选取植被覆盖严重退化和明显改善2个区域,结合TM影像和MODIS影像进行验证.同时,对植被覆盖变化的主要气候影响因素进行研究分析,以期为海河流域生态环境的恢复和治理建设提供一定的理论依据和参考.1数据与方法1.1研究区域概况海河流域位于112毅~120毅E,35毅~43毅N,流域总面积3.182伊105km2,占全国总面积的3.3%,其中山区面积占59%,平原面积占41%.海河流域总体地势西北高、东南低,属于温带半干旱季风气候区,包括海河、滦河和徒骇马颊河3大水系.1.2数据来源与处理MODIS影像数据来源于美国航空航天局(NASA)数据中心,包括EOS/Terra卫星的MODIS/NDVI产品MOD13Q1和EOS/Aqua卫星的MODIS/NDVI产品MYD13Q1.海河流域覆盖4景MODIS/NDVI数据,分别为h26v04、h26v05、h27v04和h27v05.该数据集的空间分辨率为250m,时间分辨率为16d.其中,EOS/Terra 卫星数据产品的时间刻度为2000—2016年,EOS/Aqua 卫星数据产品的时间刻度为2002—2016年.影像数据在获取过程中会受大气等因素的影响,在使用这些影像数据前需要对其进行投影转换、变换数据格式等预处理.经过预处理后的数据采用最大值合成法(maximum value composite,MVC)将2000—2016年NDVI数据逐年合成,逐像元比较NDVI值,选取最大值作为合成后的年度NDVI数据集,排除和降低大气、云和太阳高度角等因素对影像数据造成的影响[22].最终得到海河流域2000—2016年共计17年最大NDVI数据集,选取其中2000、2009和2016年3期结果如图1所示.气象数据来源于中国气象局国家气象信息中心图1海河流域2000、2009和2016年最大NDVI的空间分布Fig.1Annual maximum NDVI spatial distribution of the Haihe River Basin in2000,2009and2016(b)2009(c)2016NNN(a)2000LegendMax NDVI<00-0.250.25-0.500.50-0.75>0.7504080160240320kmLegendMax NDVI<00-0.250.25-0.500.50-0.75>0.7504080160240320kmLegendMax NDVI<00-0.250.25-0.500.50-0.75>0.7504080160240320km55··的中国地面降水[23]和气温[24]日值0.5毅伊0.5毅格点数据集.该数据集基于2472个国家级地面气象站获取的基本气象要素资料,利用薄盘样条法(thin plate spline ,TPS )进行空间插值得到中国地面(72毅~136毅E ,18毅~54毅N )空间分辨率0.5毅伊0.5毅的日值降水和气温格点数据.本研究采用的气象数据时间跨度为2000—2016年,时间分辨率为24h.将降水和气温日值数据分别进行预处理,得到2000—2016年海河流域局部区域的气温和降水数据集,选取其中2000、2009和2016年3期结果如图2所示.1.3研究方法本研究在统计海河流域NDVI 时,采用均值法计算流域内所有像元NDVI 的平均值,计算公式为I NDVI =mi=1移(移I NDVI x ,y)/nm(1)式(1)中:I NDVI 为海河流域的NDVI 平均值;x 为海河流域像元行数;y 为流域像元列数;n 为流域内像元总数;i =1,2,…,m ,本研究时间刻度为2000—2016年,对应时间跨度m 为17.趋势分析是对海河流域NDVI 时间序列影像数据进行回归分析,并预测流域内植被覆盖的变化趋势.采用一元线性回归趋势法,通过统计海河流域年最大NDVI 数据集每个像元2000—2016年的值,采用趋势分析法模拟分析该像元17年间的变化趋势,反映海河流域植被覆盖的年际变化及时空变化规律.K NDVI =m 伊m i =1移i 伊NDVI k -m i =1移i mi =1移NDVI im 伊mi =1移i 2-m i =1移i蓸蔀2(2)式(2)中:K NDVI 为每个像元NDVI 的斜率,即为海河流域2000—2016年NDVI 的年际变化趋势.i 为1~m 的年序号,本研究时间刻度为2000—2016年,对应时间跨度m 为17;NDVI i 为第i 年的NDVI 值.K NDVI >0表示海河流域植被覆盖度的变化趋势是增加的,反之则减少;K NDVI =0说明随着时间的变化,植被覆盖变化趋势不变.根据计算所得每个栅格像元的线性回归率,将计算结果分为5类:严重退化区、轻微退化区、基本不变区、轻微改善区和明显改善区,此方法可以有效区分出海河流域植被覆盖度的变化趋势.(b )Annual average precipitation(a )Annual average temperature图2海河流域2000、2009和2016年平均气温和降水量的空间分布Fig.2Spatial distribution of annual average temperature and annual average precipitation in Haihe River Basin in 2000,2009and 20160320km 24016080400320km 24016080400320km24016080400320km24016080400320km24016080400320km240160804015益10益5益0益15益10益5益0益15益10益5益0益200020092016NNNNNN2000200920161000mm 800mm 600mm 400mm 200mm 0mm1000mm 800mm 600mm 400mm 200mm 0mm1000mm 800mm 600mm 400mm 200mm 0mm56··本文采用NDVI 与气温和降水的相关系数探讨研究区气温和降水量变化对NDVI 的影响,相关系数的计算公式为r =mi=1移(x i-x )(y i-y )mi=1移(x i-x )2姨m i=1移(y i-y )2(3)式(3)中:r 代表变量x (NDVI )和y (气温或降水量)之间的相关系数,取值范围为[-1,1],其数值越大,表明2个变量间的相关性越强;m 为时间序列长度17年;i 为年份;x i 为NDVI 在第i 年的值;x 为NDVI 在17年间数值的年平均值;y i 为气温或降水量在第i 年的数值;y 为气温或降水量在17年间数值的年平均值.2结果与分析2.1NDVI 空间分布特征利用海河流域2000—2016年的年度NDVI 最大值数据集,计算得到流域17年的NDVI 平均值.根据水利部2007年颁布的《土壤侵蚀分类分级标准》[25],结合海河流域植被覆盖类型特征,将海河流域植被盖度划分为极低覆盖度、低覆盖度、中覆盖度、中高覆盖度和高覆盖度地5种覆盖类型,划分范围和特点如表1所示.表1植被覆盖度划分表Tab.1Classification of vegetation coverageCoverage degree Vegetation coverage/%CharacteristicExtremely low00-100Residents ,water areas ,bare fields ,traffic landsLow10-30Low yield of grasslands ,wastelands ,open forest landsMedium30-50Medium yield of grasslands ,croplands ,low confinement woodlandsAbove average50-70Slightly higher yield of grasslands ,forest lands ,beach wetlandsHigh70-100High yield of grasslands ,dense woodlands ,dense bushesN240km180********Extremely lowLow MediumAbove average High表22000—2016年海河流域不同植被覆盖度的面积Tab.2Different vegetation coverage area in the Haihe River Basin from 2000to 2016依据植被盖度分级分类标准,得到2000—2016年海河流域植被盖度空间分布图和海河流域2000—2016年不同植被盖度面积表,分别如图3和表2所示.由图3和表2可以看出,海河流域NDVI 值小于0.1的极低覆盖度区域主要为湿地、水域和建设用地,面积为1.7伊103km 2,占流域总面积的0.53%,主要分布在环渤海湾地区;低覆盖度区域面积为1.2伊103km 2,占流域总面积的0.38%,主要分布于城市中心和环渤海湾一带,以建设用地为主;中覆盖度NDVI 值为0.3~0.5,主要为中产草地、农田和低郁闭度林地等,面积占比3.83%;中高覆盖度NDVI 值为0.5~0.7,主要由中高产草地、林地和滩地组成,面积为5.8伊104km 2;海河流域NDVI 值大于0.7的高覆盖度区域面积较大,占流域总面积的76.93%,主要为高产草地、密林地和农田,集中分布于辽宁、山东和河南等地.综上可知,研究区植被覆盖率总体较高,仅环渤海湾地带和一些城市区域植被覆盖率较低.图32000—2016年海河流域不同植被覆盖度的空间分布Fig.3Spatial distribution of different vegetation coverage in the Haihe River Basin from 2000to 2016NDVI range CoverageArea/(伊104km 2)Proportion/%0-0.1.0Extrerndy low 00.1700.530.7-1.0High24.4876.930.1-0.3Low 0.120.380.3-0.5Medium 1.22 3.830.5-0.7Above average5.8318.3257··图52000—2016年海河流域NDVI 变化趋势Fig.5Trends of NDVI in the Haihe River Basin from2000to 2016N240km 180********Severe degradation Slight degradation InvariantSlight improvementObvious improvement表3趋势变化区域的统计数据Tab.3Statistics of trends of changed areaTrends of NDVITypeArea/(伊104km 2)Proportion/%K NDVI 臆-0.0101Severe degradation00.7002.18-0.0101约K NDVI 约-0.0016Slight degradation 3.4010.70-0.0016臆K NDVI 臆0.0034Invariant13.8543.530.0034约K NDVI 约0.0083Slight improvement10.3232.45K NDVI 逸0.0083Obvious improvement 03.5411.14Trend2.2NDVI 时序变化特征利用海河流域2000—2016年的年度NDVI 最大值数据集制作折线图并拟合出17年间NDVI 的变化趋势,得到2000—2016年海河流域NDVI 年际变化情况,结果如图4所示.由图4可以看出,海河流域NDVI 在0.70~0.79间波动,NDVI 的多年平均值为0.76,总体呈上升趋势,线性增长率为0.027/10a.其中2000—2004年,NDVI 上升趋势明显,线性增长率为0.184/10a ,植被覆盖情况有所改善;2004—2008年,NDVI 呈缓慢增长趋势,2008年出现最大值;NDVI 在2009年和2014年出现谷值,说明流域内植被覆盖有所退化;2014年后,NDVI 线性增长率为0.145/10a ,植被覆盖有所提升.综上所述,2000—2016年间海河流域植被覆盖状况的变化较为明显,17年间研究区的植被覆盖状况整体上得到较大改善.这与海河流域范围内实施的一系列生态建设工程密切相关,如三北防护林工程、退耕还林还草工程以及京津风沙源治理工程等,这些生态建设工程有效增加了海河流域地表植被的覆盖率.2.3NDVI 变化趋势分析基于2000—2016年海河流域的逐年NDVI 最大值数据集,采用一元线性回归趋势分析法计算得到海河流域NDVI 年际变化情况,K NDVI 的变化范围为-0.0610~0.0538,植被变化趋势较为明显.采用Jenks 自然间断点分级法(一种地图分级算法,类内差异最小,类间差异最大,利用数据本身有断点的特征进行聚类分级)将海河流域NDVI 年际变化分为严重退化、轻微退化、基本不变、轻微改善和明显改善共5个等级,得到2000—2016年海河流域NDVI 变化趋势以及趋势变化区域的统计数据,结果分别如图5和表3所示.由图5和表3可以看出,海河流域植被覆盖改善区域面积为1.386伊105km 2,远大于退化区域,其中改善部分以轻微改善为主,占流域总面积的32.45%;退化区域面积为4.1伊104km 2,占总面积的12.88%,环状分布于城市的周围,北京和天津最为明显;基本不变区域交错分布于改善区域和退化区域之间,面积占流域总面积的43.53%.综上可知,海河流域近17年来植被覆盖变化存在显著的空间差异性,人类活动等对地表植被覆盖所产生的正负效应同时存在,研究区大部分地区的植被得到了明显改善,但受城市扩张等因素的影响,城市外围区域的植被退化现象较为严重.2.4变化趋势结果验证为了验证一元线性回归趋势分析方法对海河流域植被覆盖进行趋势分析的可靠性,在研究区域中分别选取植被覆盖严重退化区域A 和植被覆盖明显改善区域B ,如图5中方框所示.选用TM 和MODIS 影图42000—2016年海河流域NDVI 年际变化Fig.4Inter -annual variation of NDVI in the Haihe RiverBasin from 2000to 20160.800.780.760.740.720.700.682000Year20022004200620082010201220142016y =0.0027x +0.7392R 2=0.347558··图6验证区域A 和B 对应的Landsat 和MODIS 影像Fig.6Landsat and MODIS images of the validation regions A and B(a )Large version of region A in Fig.5(c )TM (4,3,2)composite image of region A in different years(d )MODIS NDVI of region A in different years(e )TM (4,3,2)composite image of region B in different years(f)MODIS NDVI of region B in different years(b )Large version of region B in Fig.5TM2000TM2009TM2016MODIS2000MODIS2009MODIS2016MODIS2000MODIS2009MODIS2016TM2000TM2009TM2016像对区域A 和区域B 这2个植被覆盖显著变化区域进行验证,二者的NDVI 变化趋势局部放大图以及对应的TM 和MODIS NDVI 产品影像如图6所示.图6中TM 影像为4,3,2波段合成的标准假彩色影像,红色代表植被,MODIS NDVI 产品影像中颜色越深代表NDVI 值越小,即植被覆盖度越低.59··区域A 为植被退化区域,主要涵盖天津市10个区(红桥区、河北区、河东区、河西区、和平区、南开区、东丽区、津南区、西青区以及静海区);区域B 为植被改善区域,主要包括河北省张家口市怀安县和万全区.由图6(a )可以看出,植被退化区域主要集中在天津市西青区、东丽区、津南区和静海区等城镇规模迅速扩张地区,结合图6(c )和图6(d )可以看出,2000—2016年天津市城市快速发展扩张,建筑用地显著增加,土地利用情况发生变化,植被覆盖退化较为明显,NDVI 呈现显著减小趋势.由图6(b )可以看出,植被改善区域主要集中在河北省张家口市万全区的旧堡乡和北新屯乡,该区域主要为山区,位于省级园林县城、国家重点生态功能区(防风固沙)万全区和怀安县,结合图6(e )和图6(f )影像可知,2016年较2009年相比植被覆盖区域面积明显增加,NDVI 呈现显著增加趋势.验证区域的植被变化情况与趋势分析方法所得结果相符,进一步说明了该方法提取的海河流域植被变化特征具有较高的精度.2.5植被变化气候影响因素分析为了直观展现2000—2016年17年间海河流域植被覆盖度、年降水量和年平均气温的变化趋势关系,将海河流域年降水量和年平均气温气象数据分别与海河流域NDVI 年际变化进行叠加,结果如图7所示.通过计算得到海河流域2000—2016年NDVI 与年降水量相关系数为0.51,呈显著正相关,NDVI 与年平均气温相关系数为-0.20,呈负相关且相关性较弱.海河流域属于温带半干旱季风气候,相关系数表明气温对海河流域植被生长的影响较弱,降水是影响植被覆盖最显著的气候影响因素,在气温上升、降水减少的情况下,植被覆盖度会有所下降.结合图7可知,NDVI 和年降水量在2008年和2012年出现峰值,在2009年和2014年出现谷值,二者年度变化趋势表现出较高的一致性.3结论利用MODIS/NDVI 数据分析海河流域近17年植被空间分布、时序变化和空间变化特征及其与气候影响因素的关系,得到以下主要结论:(1)空间分布:通过海河流域植被盖度空间分布状况可以看出,海河流域整体植被覆盖较高,覆盖类型以高覆盖度(NDVI 跃0.7)为主,面积为2.448伊105km 2,占流域总面积的76.93%,植被类型主要为高产草地、密林地和密灌地;极低覆盖度(NDVI 约0.1)区域主要分布在环渤海湾地区,面积不足流域总面积的1%.(2)时序变化:通过海河流域植被年NDVI 可以看出,海河流域NDVI 值近17年变化范围为0.70~0.79,总体呈上升趋势,线性增长率为0.027/10a ,时序变化的多年平均值为0.76.其中2000—2008年NDVI 呈增长趋势,且在2008年达到最大值0.79;2009年和2014年出现谷值,说明流域内植被覆盖有所退化.(3)空间变化:通过海河流域近17年植被NDVI 变化趋势可以看出,海河流域植被空间变化趋势以改善为主,其中变化趋势大于0.0034小于0.0083为轻微改善,占流域总面积的32.45%;变化趋势大于0.0083为明显改善,占流域总面积的11.14%;退化区域面积占比不足13%.在植被变化趋势结果验证中,对比植被变化趋势较为明显的2个区域,验证区的变化特征与趋势分析方法所得结果表现出较好的空间一致性.(4)气候影响因素:海河流域为温带半干旱季风气候,选择年平均气温和年降水量对海河流域植被覆盖变化进行相关性分析,结果表明年平均气温与NDVI 相关性较弱,说明气温对海河流域植被生长的影响较弱;年降水量与NDVI 相关系数为0.51,呈显著正相关,说明降水是影响海河流域植被覆盖较为显著的气候影响因素.本研究利用海河流域2000—2016年MODIS/NDVI 数据计算分析了近17年海河流域植被时空分布及变化规律特征,快速准确地表达了海河流域植被生长现状及演变趋势,并对变化趋势结果进行验证.在进行植被影响因素分析时,以气温和降水作为气候影响因素对植被变化进行相关性分析,没有考虑到其他气候影响因素、自然因素和人为因素对植被变化带来的影响.因此,进一步拓展植被变化影响因素分析是今后0.800.780.760.740.720.700.68y =-0.0223x +9.6153R 2=0.1003121086420y =0.0027x +0.7392R 2=0.3475图72000—2016年海河流域NDVI 、年降水量及年平均气温变化趋势Fig.7Change trend of NDVI ,annual average precipitationand annual average temperature in the Haihe River Basin from 2000to 20162000Years20022004200620082010201220142016NDVI Annual average temperatureAnnual average precipitation y =0.0492x +4.4852R 2=0.187060··工作的重点.参考文献:[1]李树元.海河流域生态环境关键要素演变规律与脆弱性研究[D].天津:天津大学,2014.LI S Y.Research on Variation of Essential Factors and Vulnerability of Aquatic Ecosystem in Haihe Basin[D].Tianjin:Tianjin University,2014(in Chinese).[2]冯露,岳德鹏,郭祥.植被指数的应用研究综述[J].林业调查规划,2009,34(2):48-52.FENG L,YUE D P,GUO X.A review on application of normal differ-ent vegetation index[J].Forest Inventory and Planning,2009,34(2):48-52(in Chinese).[3]SELLERS P J,TUCKER C J,COLLATZ G J,et al.A revised land surface parameterization(SiB2)for atmospheric GCMS.Part域:The generation of global fields of terrestrial biophysical parameters from satellite data[J].Journal of Climate,1996,9(4):706-737. [4]那晓东,张树清,李晓峰,等.MODIS NDVI时间序列在三江平原湿地植被信息提取中的应用[J].湿地科学,2007,5(3):227-236. NA X D,ZHANG S Q,LI X F,et al.Application of MODIS NDVI time series to extracting wetland vegetation information in the Sanjiang plain[J].Wetland Science,2007,5(3):227-236(in Chinese). [5]顾娟,李新,黄春林.基于时序MODIS NDVI的黑河流域土地覆盖分类研究[J].地球科学进展,2010,25(3):317-326.GU J,LI X,HUANG C nd cover classification based on time-series MODIS NDVI data in Heihe river basin[J].Advances in Earth Science,2010,25(3):317-326(in Chinese).[6]王志慧,李世明,刘良云,等.基于MODIS NDVI时间序列的土地覆盖分层分类方法研究[J].遥感技术与应用,2013,28(5):910-919.WANG Z H,LI S M,LIU L Y,et al.Hierarchical land cover classifi-cation based on MODIS NDVI time-series[J].Remote Sensing Technol-ogy and Application,2013,28(5):910-919(in Chinese). [7]王晓江,胡尔查,李爱平,等.基于MODIS NDVI的内蒙古大青山自然保护区植被覆盖度的动态变化特征[J].干旱区资源与环境,2014,28(8):61-65.WANG X J,HU E C,LI A P,et al.Dynamic changes of vegetation coverage in Daqingshan Nature Reserve based on MODIS NDVI image [J].Journal of Arid Land Resources and Environment,2014,28(8):61-65(in Chinese).[8]张莉,陈芸芝,汪小钦.基于时序MODIS NDVI数据的长汀县植被趋势特征研究[J].福州大学学报(自然科学版),2016,44(5):661-667.ZHANG L,CHEN Y Z,WANG X Q.Vegetation trends research of Changting County based on time series of MODIS NDVI[J].Journal of Fuzhou University(Natural Science Edition),2016,44(5):661-667(in Chinese).[9]冯志敏,辛海强,李军.基于MODIS植被指数的塔河流域植被时空变化特征研究[J].测绘与空间地理信息,2017,40(2):90-94. FENG Z M,XIN H Q,LI J.Study on characteristics of land cover change of time and space in Tarim river basin using MODIS NDVI[J]. Geomatics&Spatial Information Technology,2017,40(2):90-94(inChinese).[10]徐浩杰,杨太保,曾彪.2000-2010年祁连山植被MODIS NDVI的时空变化及影响因素[J].干旱区资源与环境,2012,26(11):87-91. XU H J,YANG T B,ZENG B.Spatial-temporal changes of vegetation in Qilian Mountains from2000to2010based on MODIS NDVI data and its affecting factors[J].Journal of Arid Land Resources and Enviro-nment,2012,26(11):87-91(in Chinese).[11]成方妍,刘世梁,尹艺洁,等.基于MODIS NDVI的广西沿海植被动态及其主要驱动因素[J].生态学报,2017,37(3):788-797. CHENG F Y,LIU S L,YIN Y J,et al.The dynamics and main driving factors of coastal vegetation in Guangxi based on MODIS NDVI[J].Acta Ecologica Sinica,2017,37(3):788-797(in Chinese). [12]石悦樾,银正彤,郑文锋.基于MODIS数据乌江流域植被覆盖变化与气候变化关系研究[J].林业资源管理,2017(1):127-134. SHI Y Y,YIN Z T,ZHENG W F.Study on the response of vegetation cover change and climate change in Wujiang river basin based on MODIS data[J].Forest Resources Management,2017(1):127-134(in Chinese).[13]孙艳玲,郭鹏.1982—2006年华北植被覆盖变化及其与气候变化的关系[J].生态环境学报,2012,21(1):7-12.SUN Y L,GUO P.Variation of vegetation coverage and its relationship with climate change in North China from1982to2006[J].Ecology and Environmental Sciences,2012,21(1):7-12(in Chinese). [14]吴云,曾源,赵炎,等.基于MODIS数据的海河流域植被覆盖度估算及动态变化分析[J].资源科学,2010,32(7):1417-1424. WU Y,ZENG Y,ZHAO Y,et al.Monitoring and dynamic analysis of fractional vegetation cover in the Hai river basin based on MODIS data [J].Resources Science,2010,32(7):1417-1424(in Chinese). [15]陈福军,沈彦俊,胡乔利,等.海河流域NDVI对气候变化的响应研究[J].遥感学报,2011,15(2):401-414.CHEN F J,SHEN Y J,HU Q L,et al.Responses of NDVI to climate change in the Hai basin[J].Journal of Remote Sensing,2011,15(2):401-414(in Chinese).[16]王永财,孙艳玲,王中良.1998—2011年海河流域植被覆盖变化及气候因子驱动分析[J].资源科学,2014,36(3):594-602. WANG Y C,SUN Y L,WANG Z L.Spatial-temporal change in vege-tation cover and climate factor drivers of variation in the Haihe river basin1998-2011[J].Resources Science,2014,36(3):594-602(in Chinese).[17]杨艳丽,孙艳玲,王中良.2000—2013年海河流域植被覆盖的时空变化[J].干旱区资源与环境,2016,30(7):65-70. YANG Y L,SUN Y L,WANG Z L.The spatial-temporal variations of vegetation cover in the Haihe river basin from2000to2013[J].Journal of Arid Land Resources and Environment,2016,30(7):65-70(in Chinese).[18]陈燕丽,龙步菊,潘学标,等.MODIS NDVI和AVHRR NDVI对草原植被变化监测差异[J].遥感学报,2011,15(4):831-845. CHEN Y L,LONG B J,PAN X B,et al.Differences between MODIS NDVI and AVHRR NDVI in monitoring grasslands change[J].Journal of Remote Sensing,2011,15(4):831-845(in Chinese). [19]严晓瑜,董文杰,何勇.不同传感器数据在若尔盖湿地植被变化监61··(下转第68页)测应用中的适宜性分析[J].遥感技术与应用,2008,23(3):300-304.YAN X Y ,DONG W J ,HE Y.Analysis of the suitability from different remote sensing data on the monitoring of vegetation change in ruoergai marsh[J].Remote Sensing Technology and Application ,2008,23(3):300-304(in Chinese ).[20]韩鹏,姚娟,李天宏.3种不同数据源NDVI 的比较分析及其在延河流域的应用研究[J].应用基础与工程科学学报,2014,22(4):661-674.HAN P ,YAO J ,LI T parison of 3NDVI datasets and the appli -cation at Yanhe basin ,China[J].Journal of Basic Science and Enginee -ring ,2014,22(4):661-674(in Chinese ).[21]HUETE A ,JUSTICE C ,LEEUWEN W V.MODIS vegetation index (MOD13);algorithm theoretical basis document[EB/OL].(2013-12-31)[2019-06-10]./data/atbd_mod13.pdf.[22]宋富强,康慕谊,杨朋,等.陕北地区GIMMS 、SPOT :VGT 和MODIS 归一化植被指数的差异分析[J].北京林业大学学报,2010,32(4):72-80.SONG F Q ,KANG M Y ,YANG P ,et parison and validation of GIMMS ,SPOT -VGT and MODIS global NDVI products in the Loess Plateau of northern Shanxi Province ,northwestern China [J].Journal of Beijing Forestry University ,2010,32(4):72-80(in Chinese ).[23]国家气象科学数据中心.中国地面降水日值0.5毅伊0.5毅格点数据集(V2.0)[EB/OL].http :///data/cdcdetail/dataCode/SURF_CLI_CHN_PRE_DAY_GRID_0.5.html.National Meteorological Information Center.Dataset of 0.5毅伊0.5毅grid points of daily ground precipitation in China(V2.0)[EB/OL].http :///data/cdcdetail/dataCode/SURF_CLI_CHN_PRE_DAY_GRID_0.5.html.[24]国家气象科学数据中心.中国地面气温日值0.5毅伊0.5毅格点数据集(V2.0)[EB/OL].http :///data/cdcdetail/dataCode/SURF_CLI_CHN_TEM_DAY_GRID_0.5.html.National Meteorological Information Center.Dataset of 0.5毅伊0.5毅grid points of daily ground temperature in China (V2.0)[EB/OL].http :///data/cdcdetail/dataCode/SURF_CLI_CHN_TEM_DAY_GRID_0.5.html(in Chinese ).[25]中华人民共和国水利部.GB/SL190-2007,土壤侵蚀分类分级标准[S].北京:中国水利水电出版社,2008.Ministry of Water Resources of the People忆s Republic of China.GB/SL190-2007,Classification and Classification Standards of Soil Ero -sion[S].Beijing :China Water&Power Press ,2008(in Chinese ).(责任编校亢原彬)(上接第61页)ZOU Z C ,DENG Y C.Study on numerical simulation of wind field us -ing three kinds of Terra in data[J].Water Resources and Power ,2015,33(1):204-206,182(in Chinese ).[12]张小培,银燕.复杂地形地区WRF 模式四种边界层参数化方案的评估[J].大气科学学报,2013,36(1):68-76.ZHANG X P ,YIN Y.Evaluation of the four PBL schemes in WRF model over complex topographic areas[J].Transactions of AtmosphericSciences ,2013,36(1):68-76(in Chinese ).[13]潘小多,李新,冉有华,等.下垫面对WRF 模式模拟黑河流域区域气候精度影响研究[J].高原气象,2012,31(3):657-667.PAN X D ,LI X ,RAN Y H ,et al.Study on the influence of the under -lay on the climate accuracy of the Heihe River Basin in the face of WRFmodel[J].Plateau Meteorology ,2012,31(3):657-667(in Chinese ).[14]DANIELSON J J ,GESCH D B.Global Multi -Resolution Terrain Ele -vation Data 2010(GMTED2010)[M].U.S.Geological Survey Open -File Report 2011-1073,2011.[15]JAVIS A ,REUTER H I ,NELSON A ,et al.Hole -filled SRTM for the globe version 4[M].CGIAR -CSI SRTM 90m Database ,2008.[16]孟露,赵天良,杨兴华,等.塔克拉玛干沙漠腹地大气边界层参数化方案的模拟评估[J].气象科学,2018,38(2):157-166.MENG L ,ZHAO T L ,YANG X H ,et al.An assessment of atmospheric boundary layer schemes over the Taklimakan Desert hinterland[J].Jour -nal of the Meteorological Sciences ,2018,38(2):157-166(in Chinese ).[17]WANG Z Q ,DUAN A M ,WU G X.Impacts of boundary layer parame -terization schemes and air -sea coupling on WRF simulation of the EastAsian summer monsoon[J].Science China :Earth Sciences ,2014,57:1480-1493.[18]ATHMANIA D ,ACHOUR H.External validation of the Aster GDEM2,GMTED2010and CGIAR -CSI -SRTM v4.1free access digital elevation models (DEMs )in Tunisia and Algeria[J].Remote Sensing ,2014,6(5):4600-4620.[19]VARGA M ,BA譒I 鸳悦T.Accuracy validation and comparison of global digital elevation models over Croatia[J].International Journal of RemoteSensing ,2015,36(1):170-189.[20]董俊玲,韩志伟,张仁健,等.WRF 模式对中国城市和半干旱地区气象要素的模拟检验和对比分析[J].气象科学,2011,31(4):484-492.DONG J L ,HAN Z W ,ZHANG R J ,et al.Evaluation and analysis of WRF simulated meteorological variables in the urban and semi -arid ar -eas of China[J].Journal of the Meteorological Sciences ,2011,31(4):484-492(in Chinese ).(责任编校亢原彬)68··。
亚热带低丘区氮磷流失浓度和化学形态的季节性变化特点
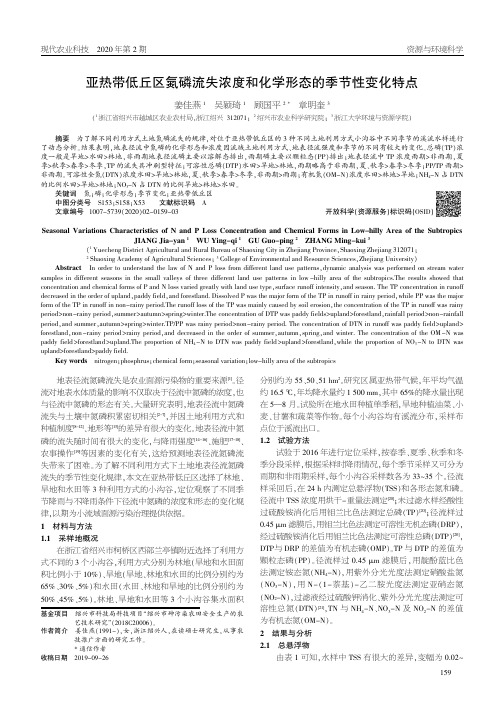
摘要为了解不同利用方式土地氮磷流失的规律,对位于亚热带低丘区的3种不同土地利用方式小沟谷中不同季节的溪流水样进行了动态分析。
结果表明,地表径流中氮磷的化学形态和浓度因流域土地利用方式、地表径流强度和季节的不同有较大的变化。
总磷(TP )浓度一般是旱地>水田>林地,非雨期地表径流磷主要以溶解态排出,雨期磷主要以颗粒态(PP )排出;地表径流中TP 浓度雨期>非雨期,夏季>秋季>春季>冬季,TP 的流失具冲刷型特征;可溶性总磷(DTP )水田>旱地>林地,雨期略高于非雨期,夏、秋季>春季>冬季;PP/TP 雨期>非雨期。
可溶性全氮(DTN )浓度水田>旱地>林地,夏、秋季>春季>冬季,非雨期>雨期;有机氮(OM-N )浓度水田>林地>旱地;NH 4-N 占DTN 的比例水田>旱地>林地;NO 3-N 占DTN 的比例旱地>林地>水田。
关键词氮;磷;化学形态;季节变化;亚热带低丘区中图分类号S153;S158;X53文献标识码A 文章编号1007-5739(2020)02-0159-03开放科学(资源服务)标识码(OSID )Seasonal Variations Characteristics of N and P Loss Concentration and Chemical Forms in Low-hilly Area of the SubtropicsJIANG Jia-yan 1WU Ying-qi 1GU Guo-ping 2ZHANG Ming-kui 3(1Yuecheng District Agricultural and Rural Bureau of Shaoxing City in Zhejiang Province ,Shaoxing Zhejiang 312071;2Shaoxing Academy of Agricultural Sciences ;3College of Environmental and Resource Sciences ,Zhejiang University )Abstract In order to understand the law of N and P loss from different land use patterns ,dynamic analysis was performed on stream water samples in different seasons in the small valleys of three different land use patterns in low -hilly area of the subtropics.The results showed that concentration and chemical forms of P and N loss varied greatly with land use type ,surface runoff intensity ,and season.The TP concentration in runoff decreased in the order of upland ,paddy field ,and forestland.Dissolved P was the major form of the TP in runoff in rainy period ,while PP was the major form of the TP in runoff in non-rainy period.The runoff loss of the TP was mainly caused by soil erosion ,the concentration of the TP in runoff was rainy period >non-rainy period ,summer >autumn >spring >winter.The concentration of DTP was paddy fields >upland >forestland ,rainfall period >non-rainfall period ,and summer ,autumn >spring >winter.TP/PP was rainy period >non-rainy period.The concentration of DTN in runoff was paddy field >upland >forestland ,non -rainy period >rainy period ,and decreased in the order of summer ,autumn ,spring ,and winter.The concentration of the OM -N was paddy field >forestland >upland.The proportion of NH 4-N to DTN was paddy field >upland >forestland ,while the proportion of NO 3-N to DTN was upland >forestland >paddy field.Key words nitrogen ;phosphrus ;chemical form ;seasonal variation ;low-hilly area of the subtropics亚热带低丘区氮磷流失浓度和化学形态的季节性变化特点姜佳燕1吴颖琦1顾国平2*章明奎3(1浙江省绍兴市越城区农业农村局,浙江绍兴312071;2绍兴市农业科学研究院;3浙江大学环境与资源学院)地表径流氮磷流失是农业面源污染物的重要来源[1]。
季节差异 英语阅读

季节差异英语阅读The Embrace of Seasonal Differences.As the world turns on its axis, each passing day ushers in a new tale of the natural world, a story that is often told through the changing seasons. The四季, each with its unique character and beauty, play a pivotal role in shaping the very essence of life on Earth. From the blossoms of Spring to the crisp chill of Winter, each season brings a fresh perspective, a new way of understanding the world around us.Spring: A Time of Renewal.Spring is the epitome of rebirth, a time when nature comes alive with a burst of energy and color. As the snow melts and flowers bloom, the air fills with the scent of fresh soil and blooming flowers. Trees burst into leaf, and birds sing their jubilant songs, marking the end of dormancy and the beginning of a new cycle of life. It is atime of hope and optimism, a time when the world seems to be saying, "Anything is possible."Summer: The Vigor of Life.Summer is the season of full bloom, when the sun shines brightly and the world is abuzz with activity. The heat of the sun brings out the vibrant hues of nature, from the deep green of lush foliage to the rich hues of fruits and flowers. It is a time for fun and frolic, for outdoor adventures and lazy afternoons spent in the shade. The energy and vivacity of summer are a testament to the resilience and vitality of life.Autumn: A Time of Tranquility.Autumn, often referred to as the "harvest season," is a time of rich rewards and serene beauty. As the leaves turn golden and red, the air fills with the scent of falling leaves and the promise of a new beginning. It is a time for reflection and introspection, for looking back on the year gone by and looking forward to what lies ahead. Thetranquility of autumn is a balm for the soul, a reminderthat even in the midst of change, there is a sense of peace and harmony.Winter: The Embrace of Silence.Winter is the season of stillness and silence, a time when nature takes a break and the world seems to go into a deep sleep. The snow covers everything in a blanket of white, muffling the sounds and slowing down the pace oflife. It is a time for warmth and comfort, for curling up with a good book and a cup of hot cocoa. The silence of winter is not a lack of life, but a deep, abiding stillness that is both calming and meditative.The四季, each with its own unique character and beauty, are an integral part of the natural cycle of life. They teach us about the importance of change, of accepting and embracing the different stages of life. As the seasons change, so do we, growing and evolving with each passing day. Let us cherish the embrace of seasonal differences,and find joy and peace in the beauty that each season brings.。
211188330_腾格里沙漠南缘不同恢复阶段沙质草地植被的季节变化特征
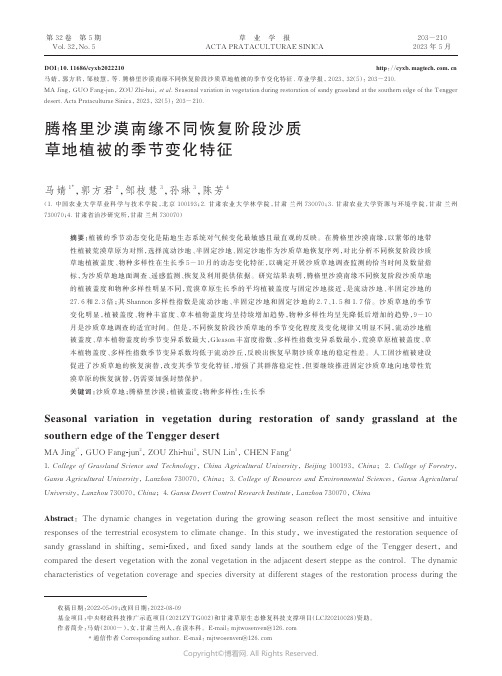
第 32 卷第 5 期Vol.32,No.5203-2102023 年 5 月草业学报ACTA PRATACULTURAE SINICA马婧,郭方君,邹枝慧,等. 腾格里沙漠南缘不同恢复阶段沙质草地植被的季节变化特征. 草业学报, 2023, 32(5): 203−210.MA Jing, GUO Fang-jun, ZOU Zhi-hui,et al. Seasonal variation in vegetation during restoration of sandy grassland at the southern edge of the Tengger desert. Acta Prataculturae Sinica, 2023, 32(5): 203−210.腾格里沙漠南缘不同恢复阶段沙质草地植被的季节变化特征马婧1*,郭方君2,邹枝慧3,孙琳3,陈芳4(1.中国农业大学草业科学与技术学院,北京 100193;2.甘肃农业大学林学院,甘肃兰州 730070;3.甘肃农业大学资源与环境学院,甘肃兰州730070;4.甘肃省治沙研究所,甘肃兰州 730070)摘要:植被的季节动态变化是陆地生态系统对气候变化最敏感且最直观的反映。
在腾格里沙漠南缘,以紧邻的地带性植被荒漠草原为对照,选择流动沙地、半固定沙地、固定沙地作为沙质草地恢复序列,对比分析不同恢复阶段沙质草地植被盖度、物种多样性在生长季5-10月的动态变化特征,以确定开展沙质草地调查监测的恰当时间及数量指标,为沙质草地地面调查、遥感监测、恢复及利用提供依据。
研究结果表明,腾格里沙漠南缘不同恢复阶段沙质草地的植被盖度和物种多样性明显不同,荒漠草原生长季的平均植被盖度与固定沙地接近,是流动沙地、半固定沙地的27.6和2.3倍;其Shannon多样性指数是流动沙地、半固定沙地和固定沙地的2.7、1.5和1.7倍。
沙质草地的季节变化明显,植被盖度、物种丰富度、草本植物盖度均呈持续增加趋势,物种多样性均呈先降低后增加的趋势,9-10月是沙质草地调查的适宜时间。
关于南极洲的知识英语作文
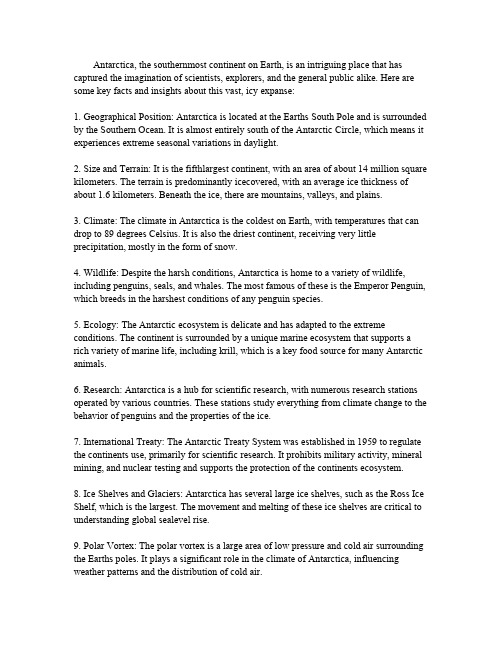
Antarctica,the southernmost continent on Earth,is an intriguing place that has captured the imagination of scientists,explorers,and the general public alike.Here are some key facts and insights about this vast,icy expanse:1.Geographical Position:Antarctica is located at the Earths South Pole and is surrounded by the Southern Ocean.It is almost entirely south of the Antarctic Circle,which means it experiences extreme seasonal variations in daylight.2.Size and Terrain:It is the fifthlargest continent,with an area of about14million square kilometers.The terrain is predominantly icecovered,with an average ice thickness of about1.6kilometers.Beneath the ice,there are mountains,valleys,and plains.3.Climate:The climate in Antarctica is the coldest on Earth,with temperatures that can drop to89degrees Celsius.It is also the driest continent,receiving very little precipitation,mostly in the form of snow.4.Wildlife:Despite the harsh conditions,Antarctica is home to a variety of wildlife, including penguins,seals,and whales.The most famous of these is the Emperor Penguin, which breeds in the harshest conditions of any penguin species.5.Ecology:The Antarctic ecosystem is delicate and has adapted to the extreme conditions.The continent is surrounded by a unique marine ecosystem that supports a rich variety of marine life,including krill,which is a key food source for many Antarctic animals.6.Research:Antarctica is a hub for scientific research,with numerous research stations operated by various countries.These stations study everything from climate change to the behavior of penguins and the properties of the ice.7.International Treaty:The Antarctic Treaty System was established in1959to regulate the continents use,primarily for scientific research.It prohibits military activity,mineral mining,and nuclear testing and supports the protection of the continents ecosystem.8.Ice Shelves and Glaciers:Antarctica has several large ice shelves,such as the Ross Ice Shelf,which is the largest.The movement and melting of these ice shelves are critical to understanding global sealevel rise.9.Polar Vortex:The polar vortex is a large area of low pressure and cold air surrounding the Earths poles.It plays a significant role in the climate of Antarctica,influencing weather patterns and the distribution of cold air.10.Mystery and Exploration:Antarctica remains a place of mystery and exploration. While much is known about its geography and wildlife,there is still much to learn about its geology,climate history,and the potential for life beneath its ice.In conclusion,Antarctica is a continent of extremes,offering a unique environment for scientific study and a stark reminder of the power and resilience of life in the most inhospitable conditions on our planet.。
英语作文校服设计
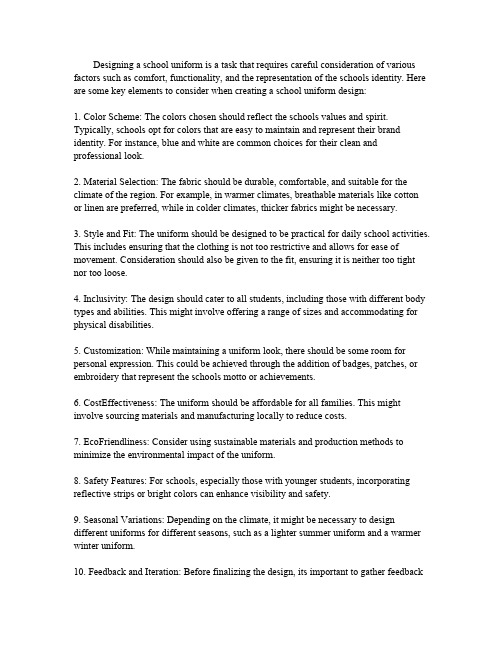
Designing a school uniform is a task that requires careful consideration of various factors such as comfort,functionality,and the representation of the schools identity.Here are some key elements to consider when creating a school uniform design:1.Color Scheme:The colors chosen should reflect the schools values and spirit. Typically,schools opt for colors that are easy to maintain and represent their brand identity.For instance,blue and white are common choices for their clean and professional look.2.Material Selection:The fabric should be durable,comfortable,and suitable for the climate of the region.For example,in warmer climates,breathable materials like cotton or linen are preferred,while in colder climates,thicker fabrics might be necessary.3.Style and Fit:The uniform should be designed to be practical for daily school activities. This includes ensuring that the clothing is not too restrictive and allows for ease of movement.Consideration should also be given to the fit,ensuring it is neither too tight nor too loose.4.Inclusivity:The design should cater to all students,including those with different body types and abilities.This might involve offering a range of sizes and accommodating for physical disabilities.5.Customization:While maintaining a uniform look,there should be some room for personal expression.This could be achieved through the addition of badges,patches,or embroidery that represent the schools motto or achievements.6.CostEffectiveness:The uniform should be affordable for all families.This might involve sourcing materials and manufacturing locally to reduce costs.7.EcoFriendliness:Consider using sustainable materials and production methods to minimize the environmental impact of the uniform.8.Safety Features:For schools,especially those with younger students,incorporating reflective strips or bright colors can enhance visibility and safety.9.Seasonal Variations:Depending on the climate,it might be necessary to design different uniforms for different seasons,such as a lighter summer uniform and a warmer winter uniform.10.Feedback and Iteration:Before finalizing the design,its important to gather feedbackfrom students,parents,and staff.This can help identify any issues or preferences that were not initially considered.11.Supplier Partnership:Collaborate with a reliable supplier who can deliver the uniforms according to the design specifications and in a timely manner.12.Regulation Compliance:Ensure that the uniform design complies with any local or national regulations regarding school uniforms.By taking these factors into account,a school can create a uniform that is not only visually appealing but also functional and representative of the schools ethos.。
- 1、下载文档前请自行甄别文档内容的完整性,平台不提供额外的编辑、内容补充、找答案等附加服务。
- 2、"仅部分预览"的文档,不可在线预览部分如存在完整性等问题,可反馈申请退款(可完整预览的文档不适用该条件!)。
- 3、如文档侵犯您的权益,请联系客服反馈,我们会尽快为您处理(人工客服工作时间:9:00-18:30)。
Atmos.Chem.Phys.Discuss.,10,7079–7113,2010 /10/7079/2010/©Author(s)2010.This work is distributed underthe Creative Commons Attribution3.0License.Atmospheric Chemistry and Physics DiscussionsThis discussion paper is/has been under review for the journal Atmospheric Chemistry and Physics(ACP).Please refer to the correspondingfinal paper in ACP if available. Seasonal variations and spatial distribution of carbonaceous aerosolsin TaiwanC.C.-K.Chou1,C.T.Lee2,M.T.Cheng3,C.S.Yuan4,S.J.Chen5,Y.L.Wu6,W.C.Hsu7,S.C.Lung1,S.C.Hsu1,C.Y.Lin1,and S.C.Liu11Research Center for Environmental Changes,Academia Sinica,Taipei,Taiwan2Institute of Environmental Engineering,National Central University,Chungli,T aiwan3Department of Environmental Engineering,National Chung Hsing University,T aichung,Taiwan4Institute of Environmental Engineering,National Sun Y at-Sen University,Kaohsiung,Taiwan 5Department of Environmental Science and Engineering,National Pingtung University of Science and Technology,Pingtung,Taiwan6Department of Environmental Engineering,National Cheng Kung University,T ainan,Taiwan70797Department of Environmental Resources Management,Dahan Institute of Technology, Hualien,TaiwanReceived:2March2010–Accepted:8March2010–Published:16March2010 Correspondence to:C.C.-K.Chou(ckchou@.tw)Published by Copernicus Publications on behalf of the European Geosciences Union.AbstractTo investigate the physico-chemical properties of aerosols in T aiwan,an observation network was initiated in2003.In this work,the measurements of the mass concen-tration and carbonaceous composition of PM10and PM2.5are presented.Analysis on the data collected in thefirst5-years,from2003to2007,showed that there was a 5very strong contrast in the aerosolfield between the rural and the urban/suburban sta-tions.Thefive-year means of EC at the respective stations ranged from0.9±0.04to4.2±0.1µgC m−3.In rural areas,EC accounted for2–3%of PM10and3–5%of PM2.5mass loadings,comparing to4–6%of PM10and4–8%of PM2.5in the urban areas.Itwas found that the spatial distribution of EC was consistent with CO and NO x across the 10network stations,suggesting that the levels of EC over T aiwan were dominated by local sources.The measured OC was split into POC and SOC counterparts following the EC tracer method.Five-year means of POC ranged from1.8±0.1to9.7±0.2µgC m−3 among the stations.It was estimated that the POM contributed5–17%of PM10and7–18%of PM2.5in T aiwan.On the other hand,thefive-year means of SOC ranged from 151.5±0.1to3.8±0.3µgC m−3.The mass fractions of SOM were estimated to be9–19%in PM10and14–22%in PM2.5.The results showed that the SOC did not exhibit signifi-cant urban-rural contrast as did the POC and EC.A significant cross-station correlation between SOC and total oxidant was observed,which means the spatial distribution ofSOC in T aiwan was dominated by the oxidant mixing ratio.Besides,correlation was 20also found between SOC and particulate nitrate,implying that the precursors of SOA were mainly from local anthropogenic sources.In addition to the spatial distribution,the carbonaceous aerosols also exhibited distinct seasonality.In northern T aiwan,the con-centrations of all the three carbonaceous components(EC,POC,and SOC)reachedtheir respective minima in the fall season.POC and EC increased drastically in win-25ter and peaked in spring,whereas the SOC was characterized by a bimodal pattern with the maximal concentration in winter and a second mode in summertime.In south-ern Taiwan,minimal levels of POC and EC occurred consistently in summer and the7081maxima were observed in winter,whereas the SOC peaked in summer and declined in wintertime.The discrepancies in the seasonality of carbonaceous aerosols between northern and southern Taiwan were most likely caused by the seasonal meteorological settings that dominated the dispersion of air pollutants.Moreover,it was inferred thatthe Asian pollution outbreaks could have shifted the seasonal maxima of air pollutants 5from winter to spring in the northern Taiwan,and that the biogenic SOA precursors were responsible to the elevated SOC concentrations in summer.1IntroductionIt is recognized that atmospheric aerosols have significant influences on the radiativebudget of the earth-atmosphere system and,consequently,could have substantial im-10pacts upon the climate(Kanakidou et al.,2005;IPCC,2007and references therein).Besides,a number of studies indicated consistently that exposures to airborne par-ticulate matters could result in adverse effects on public health,particularly on the respiratory and cardiovascular systems(e.g.,Davidson et al.,2005;Pope and Dock-ery,2006;Lippmann,2007).Despite the well established qualitative understanding,to 15date,a precise quantitative assessment of the aerosol effects is hard to achieve be-cause the concentration and composition,and hence the physico-chemical properties of atmospheric aerosols are highly variable over different spatial and temporal scales.Carbonaceous species are among the major constituents of atmospheric aerosols.However,the evaluation of the environmental and health impacts of the carbonaceous 20aerosols is still subject to significant uncertainties.In addition to the spatial and tempo-ral variations,the major difficulties are arisen also from the complex nature of the com-position.Carbonaceous aerosols are composed of a vast number of compounds that have not been totally resolved even using the most state-of-art instrument(Hallquist etal.,2009and references therein).Consequently,most of the investigations reported 25the measurements in terms of“Organic Carbon(OC)”and“Elemental Carbon(EC)”, which are not adequate to infer the molecular constitution of the carbonaceous speciesin the aerosols and hence prevent us from predicting and depicting their properties and behavior according to the classic chemistry and physics.The difficulties are also due to the complexity of the formation mechanisms and the emission profiles.The EC in aerosols is produced by incomplete combustion processes,which include anthro-pogenic sources such as fossil fuel combustion and natural sources like forestfires 5(McMurry et al.,2004;Hu et al.,2009).The sources of OC are even more com-plicated,which could be emitted directly from specific sources into the atmosphere or produced from the reactions of precursor hydrocarbons.Accordingly,the organic aerosols are usually divided into“Primary Organic Aerosols(POA)”and“SecondaryOrganic Aerosols(SOA)”.In urban areas,the POA are believed to be mostly from the 10exhaust of vehicles,whereas biomass burning was suggested as the predominant POA source on global scale(Hallquist et al.,2009).In contrast to the relatively clear picture of POA sources,the production of SOA is likely one of the most complicated issues in atmospheric chemistry as the reaction mechanisms and the precursors have not beenfully understood or discovered(Donahue et al.,2009).15Quantification of the concentration of SOC and/or SOA has been a challenge for years.The core issue is that the mass of SOA can not be measured directly.In the last two decades,several alternative methods were developed to estimate the amount of SOC/SOA based on the measurements of the gaseous and/or particulate tracers(T urpin and Huntzicker,1995;Schauer,1996,2002;T akegawa et al.,2006;Szidat et 20al.,2006;Gelencser et al.,2007;Docherty et al.,2008;Blanchard et al.,2008).These investigations consistently suggested that SOA constituted a considerable portion of the particulate matters in the atmosphere.Meanwhile,smog chamber studies on the formation of SOA from specific precursors have significantly improved our understand-ings of the physical and chemical processes responsible for SOA production(Odum et 25al.,1996;Forstner et al.,1997;Klotz et al.,1998;Jang and Kamens,1999;Cocker et al.,2001;Iinuma et al.,2004;Edney et al.,2005;Li et al.,2007;Sato et al.,2007).The results of these laboratory experiments provide a framework to describe SOA produc-tion mechanisms and allow the theoretical calculation of the yields of particular SOA7083compounds(Jenkin,2004;Russell and Allen,2005;Ng et al.,2006;Presto and Don-ahue,2006;Pathak et al.,2007;Saathoffet al.,2009).However,most of thefield studies indicated that the contribution of SOA to the mass loading of total aerosols were significantly larger than as expected(e.g.,Johnson et al.,2006;Simpson et al.,2007).The discrepancies between simulated and observed levels of SOA could be 5attributed to problems in the parameterization of SOA formation mechanisms,ignoring some precursors,and/or missing some sources.Besides,on the other hand,it should be noted that the evaluation of SOA models could also suffer from the uncertainties in the indirectly“measured”SOA concentrations.Taiwan is a densely populated and industrialized area and,consequently,there are 10substantial emissions of anthropogenic air pollutants over the island.Furthermore, Taiwan is located under the lee side of the East-Asian winter monsoons;the particu-late pollutants originating in the Asian continent,in particular the eastern China,could be transported to Taiwan on the northeasterlies of the monsoons,which could in turnincrease the concentration and influence the composition of the ambient aerosols.Al-15though the impacts of particulate matters are recognized,there was not a representa-tive long-term dataset of the aerosol composition in Taiwan until recent years(Chang et al.,2010).The Taiwan Aerosol Observation Network was organized to study the formation and transport mechanisms of the atmospheric aerosols.In this work,themeasurements of OC and EC from2003to2007are presented.The EC tracer method 20was applied to divide the OC into primary and secondary counterparts.Seasonal vari-ations and spatial distribution of the carbonaceous components in PM10and PM2.5 were analyzed and discussed in details.2Experimental methodsTo investigate the physical and chemical properties of aerosols in T aiwan,a sampling 25network consisting of7stations was initiated in2003.Figure1illustrates the geograph-ical locations of the respective stations.In summary,there are two rural stations andfive urban/suburban stations.The Cape Fuguei is located at the northern tip of T aiwan and Penghu is on a small isle in the Taiwan Strait,which is ca.65km offthe western coast of Taiwan.The urban/suburban stations T aipei,T aichung,T ainan,and Pingtung line in the western plains where most of the people live and are highly developed and industrialized.Hualien is a small city by the eastern coast of T aiwan and the station is 5ca.10km north from the downtown.Since the spring of2003,regular PM10/PM2.5sampling has been conducted at the network stations during the last week of each month.At times some episode-based sampling activities were also arranged.The sampling period of each sample was usu-ally12h:daytime samples were collected from08:00to20:00(LST=GMT+08:00), 10and nighttime sampling was from20:00to08:00(LST).Sometimes the sampling was conducted on daily base,i.e.from08:00to08:00of the next day.Thus,in the fol-lowing data analysis,all the half-day measurements will be paired to assimilate to the daily measurements.Those unpaired data(i.e.without the day/night counterpart)arethereby not included in this study.15Mass concentration of PM10/PM2.5was determined by gravimetric measurement of the samples collected on PTFEfilters.The PTFEfilter samples were then used for the analysis of soluble ions,which is not the subject of this study and is presented else-where(e.g.,Chou et al.,2008).The samples for carbonaceous analysis were collectedon quartzfiberfilters.Before sampling,all thefilters were baked under900◦C for3h 20to remove organic boratory test showed that the blank level of total carbon of the pre-firedfilters is below the detection limit of the analyzer.The mass loadings of Organic Carbon(OC)and Elemental Carbon(EC)on thefilter samples were analyzed using a DRI-2001A carbonaceous aerosol analyzer,following the IM-PROVE Thermo-Optical Reflectance(TOR)protocol(Chow et al.,2001).The analyzer 25was calibrated periodically using KHP standards.Moreover,daily quality check of the instrument was carried out using blankfilter and900ppm-C KHP spike.The criterion of the recovery of the900ppm-C KHP spike was set as from0.95to1.05,i.e.allowing an error of5%.The detection limit of the analyzer was determined as3times the standard7085deviation of the signals of10blankfilters,which thereby included the uncertainties due tofilter effects in addition to that of the instrument itself.Given an effectivefilter area of10.68cm2and the nominal sampling volume of10liter-per-minute,the total carbon detection limit of a24h sample is0.2µgC m−3.3Estimation of secondary organic aerosols5In this section,the organic carbon is split into primary(POC)and secondary(SOC) counterparts following the EC tracer method.Because this approach has been dis-cussed and applied in a number of papers(e.g.,T urpin and Huntzicker,1995;Russell et al.,2004),only a brief description is given here.The fundamental assumption of the EC tracer method is that the amount of POC is 10linearly proportional to EC in the aerosols:POC=α·EC+β.(1) Turpin and Huntzicker(1995)attributed theα·EC to the POC emitted together with the EC from combustion sources,and suggested the intercept,β,being the amount ofPOC generated from non-combustion sources.15In turn,the SOC is determined by subtracting the POC from the total OC:SOC=OC total−POC.(2) The majorflaw in this approach is that the scaling factor,α,is a source-specific pa-rameter.Starting from an emission inventory,one can estimate the amount of POCfrom each single source if the emission rate of EC and the POC/EC ratio are available. 20Unfortunately,it is not such a simple way for afilter sample of aerosols.The particulate matters collected on afilter are usually coming from a wide range of sources.There-fore,one cannot apply Eq.(1)directly to determine the POC unless the source appor-tionment of EC has been resolved and the POC/EC ratios of the respective sources areknown(e.g.,Gray and Cass,1986).The other possibility is that the source mix does 25not change temporally and,consequently,a characteristicαvalue can be available fora specific area.In practice,the characteristicα(i.e.POC/EC ratio)of a given area isusually derived from the measurements of OC and EC on days with low photochemical activity Russell et al.,2004;Polidori et al.,2006).The underlying assumption of this approach is that the day-to-day variations in the emission profiles of POC and 5EC are negligible,in spite of the pronounced diurnal cycles for the traffics.Obviously, successful application of the EC tracer method depends on the representativeness of the POC/EC ratio.In this study,given the benefits of a large data set accumulated over5years,thedistribution of OC/EC of each sampling site can be analyzed statistically.The data 10with OC/EC ratio less than the10th percentile,i.e.the left tail of the distribution,were selected and assumed being dominated by primary emissions.Linear regression was in turn applied to these data and the relationship between POC and EC was formulated.Figures2to3illustrate the OC/EC distributions and the regression analyses of PM10and PM2.5measurements,respectively,for the network stations.The values ofαand 15βfor each site are summarized in T able1.Theαvalues of PM10ranged from1.43to1.85in the western T aiwan(including Penghu),whereas an obviously high value of2.36turned out for Hualien station.This could be a result of the differences in source profile;Hualien is a small town and has less diesel vehicles than in the highly developed West-Taiwan.Note that significantβvalues were found in the central and southern T aiwan, 20the major agricultural areas of Taiwan,which indicates that the contributions of non-combustion sources to the organic aerosols were substantial in those areas.Theαvalues of PM2.5differ slightly from those of PM10,reflecting that the carbonaceous species existed mostly in thefine aerosols.The causes of the negativeβfor T aipei arenot clear yet,likely due to uncertainties in the sampling and statistics processes.2570874Contributions of carbonaceous species to the aerosol mass loadingsGiven the regression parameters listed in Table1,one can estimate the levels of POC and SOC,respectively,in each aerosol sample.T able2summarizes the descriptive statistics of the annual means of EC,POC,and SOC for all the network stations from2003to2007.During thefive years,there were obvious inter-annual variations in 5the concentrations of carbonaceous aerosols in T aiwan.However,the data did not show significant inter-annual trends in the mean levels of EC,POC,and SOC at all the stations.Nevertheless,it should be noted that a5-year dataset could not be enough to infer a long-term trend.Among the network stations,Penghu had the lowest levels of the carbonaceous 10species in aerosols.Five-year means(±1standard error)of EC,POC and SOC were0.9±0.04,1.8±0.1,and1.5±0.1µgC m−3,respectively,in PM10,with ca.65%of OCand ca.90%of EC confined infine aerosols(i.e.PM2.5).At the Cape Fuguei,another rural station of the network,the mean levels of EC,POC and SOC were1.5±0.1,2.5±0.1,and2.3±0.1µgC m−3,respectively,in PM10,and were1.3±0.1,2.2±0.1, 15and1.6±0.1µgC m−3,respectively,in PM2.5.The concentrations of carbonaceous aerosols in the urban/suburban areas were much higher than at the rural sites.In the western T aiwan,the mean EC(in PM10)concentrations ranged from2.7±0.1(Ping-tung)to4.2±0.1(T aichung)µgC m−3;POC(in PM10)ranged from5.6±0.2(T aipei)to9.7±0.2(Pingtung)µgC m−3;SOC(in PM10)ranged from2.0±0.2(T ainan)to3.8±0.3 20(T aichung)µgC m−3.The urban-rural contrast was even more pronounced in PM2.5.The aerosol concentration in the eastern T aiwan was significantly lower than in the western plains,agreeing with the reduced traffic and industrial emissions.However,it is remarkable that the SOC concentration in Hualien was comparable with those in thewestern T aiwan.This inconsistency between the primary and secondary components 25of the carbonaceous aerosols suggests that there could be substantial emissions of biogenic precursors for the production of secondary organic aerosols in the eastern Taiwan.The spatial distribution of SOC will be discussed in more details later on.Figures4and5illustrate the5-year mean mass concentrations and pie shares of EC,Primary Organic Matters(POM),and Secondary Organic Matters(SOM)in PM10 and PM2.5,respectively,over Taiwan.Despite the complicated composition of organic aerosols,it is generally assumed that the mass ratio of organic aerosols to the asso-ciated OC could range from1.2to1.4in urban areas.However,some more recent 5studies suggested that the secondary organic aerosols are composed of highly oxi-dized species and the mass ratio of SOM/SOC may be significantly higher than that of POM/POC(e.g.,Aiken et al.,2008;Donahue et al.,2009and references cited therein).Turpin and Lim(2001)suggested a ratio of1.3for“less water soluble”organics and3.2for“more water soluble”organics.In this work,we further extended the assumptions of 10Turpin and Lim(2001)and applied different mass conversion factors to the calculation of SOM and POM.Considering that the POM are composed mostly of insoluble com-pounds and,in contrast,the oxidation products of photochemical reactions are usually soluble in water,the mass of POM was assumed being1.3times POC,whereas SOMwas estimated as3.2times the SOC concentrations.15The strong contrast between the urban and the rural sites is obvious in Figs.4to5.At the Cape Fuguei and Penghu,the PM10and PM2.5comprised only2–3%and3–5% EC,respectively.However,in the urban areas,the EC fractions increased to4–6%for PM10,and4–8%for PM2.5.The spatial distribution of POM also showed the inherenturban-rural contrast clearly.POM contributed5–7%and7–10%to the mass of PM10 20and PM2.5,respectively,at rural sites,and increased by a factor of2–3in the urban areas.Thus,in total,the primary carbonaceous aerosols accounted for7–10%and 10–15%of the PM10and PM2.5in the rural areas,respectively,comparing to17–23% and21–25%in the urban areas.On the contrary,SOM did not exhibit such a contrast.For instance,the contribution of SOM to the aerosol mass loading at the Cape Fuguei 25was even higher than in the Metropolis Taipei(14%vs.11%for PM10;18%vs.16%for PM2.5).Moreover,in contrast to the lowest PM10and PM2.5levels among the stations, the SOM contribution was highest in Hualien that reached19%to PM10and22%to PM2.5.7089The major implication of the observed urban-rural contrast in the carbonaceous aerosols’concentrations is that the influences of the primary aerosols(e.g.,EC and POM)should be confined on urban scales and hence highly heterogeneous over a region.Although the long-range transport of aerosols from mega-cities to the remoteareas indeed occurred,the dry/wet deposition and dispersion processes along the 5transport could have reduced the aerosol concentrations significantly.Consequently, the impacts of the primary aerosols on either the public health or the solar irradiances should have a very strong gradient declining from the megacities to their surrounding rural areas.This is particularly an issue of regional climate because the aerosols couldthereby cause significant differences in the surface irradiance and,in turn,could have 10disturbed the local circulation of air mass.The influences of SOA seem being more homogeneous spatially.However,the production of SOA is much more complicated than the emission of primary aerosols.The molecular composition of the SOA in rural areas could be essentially different from that in urban areas,despite the mass concen-trations were comparable.Thus,in this context,the contribution of the anthropogenic 15precursors in urban plumes to the production of SOA in the downwind areas cannot be estimated based merely on the traditional OC/EC measurement.5Seasonal variations of the carbonaceous aerosolsTo depict the seasonality of the carbonaceous aerosols in T aiwan,the dataset wasreanalyzed in terms of seasonal mean and the results are listed in T able3.Figure6 20illustrates the seasonal variations in the mass concentrations of PM10and the asso-ciated carbonaceous components,respectively,at the network stations.Because the carbonaceous aerosols were mostly confined in thefine mode,the seasonal features of the carbonaceous constituents of PM2.5are similar with those of PM10and,therefore,are not illustrated here.The seasonal means of CO and the daily maximal1-h oxi-25dant(estimated by O3+NO2)mixing ratios,which were respectively used as indicatorsof primary and secondary pollution,are also shown in Fig.6to facilitate the following discussion.The stations in northern Taiwan(Cape Fuguei and T aipei)showed consistent sea-sonality in the concentrations of OC(both POC and SOC)and EC.The concentrations of all the three components reached their respective minima in the fall season and, 5then,increased drastically in the wintertime.The autumn minima of the carbonaceous aerosols were consistent with CO and Ox max,but disagreed with the PM10levels.The reduced level of air pollution in the fall season in T aipei was most likely a con-sequence of meteorological conditions that favored dispersion of air pollutants.Thedisagreement between PM10and CO(and EC)suggests probable contribution from 10non-combustion sources.Chou et al.(2008)reported that sea-salt and dust particles contributed a large portion of the aerosol mass in northern T aiwan during the periods of northeasterly monsoons that started from autumn.Except SOC,all the species presented in Fig.6a–b showed spring maxima.Notethat,in summertime,the primary pollutants’concentrations decreased significantly 15from their respective spring maxima,whereas the secondary species remained or even increased slightly.In fact,SOC exhibited a bimodal pattern with the maximum in winter and the second mode in summer.The high SOC levels observed in the northern T aiwan in summertime were consistent with the elevated oxidant levels,implying that the in-situ photochemical production of secondary organic aerosols was prevailing.However, 20the occurrence of the winter maxima was contradictory in this context.Given that the UV irradiance over the subtropics is still enough to support substantial photochemical reactions in wintertime,the drastic increases in the SOC concentrations from autumn to winter was probably a result of declined ambient temperature.Strader et al.(1999)showed that the partition between gas and aerosol phases of SOA compounds can 25be influenced by the ambient temperature.Statistics over1971–2000shows that the monthly average temperature in Taipei ranged from15.8◦C in January to29.2◦C in July,just being coincident with the temperature window where the SOA phase partition is most sensitive to temperature.7091In contrast to the spring maxima observed at the two northern sites,the other5sta-tions over the central and southern Taiwan exhibited winter maxima in PM10,CO,EC, and POC(shown in Fig.6c–g).The winter maxima are typical in terms of pollution me-teorology;the shallow mixing layer and the formation of surface inversion could haveinhibited the dispersion of air pollutants in that season.Thus the elevated levels of 5the PM10,CO,and oxidant in northern Taiwan during springtime need to be explained.Recent investigations showed that the transport of air pollutants originating in China could have increased the concentrations of air pollutants in the region of Northwest-ern Pacific(e.g.,Hoell et al.,1997;Jacob et al.,2003;Huebert et al.,2003).Lin etal.(2005)further showed that the influences of the Asian outflows on the air quality in 10Taiwan were declining from north to south.Moreover,observational based modeling studies showed that the Asian pollution outflow was most prevailing in springtime(Liu et al.,2003).Accordingly,it was inferred that the long-range transport of air pollutants from China could have shifted the maxima of the concentrations of air pollutants fromwinter to spring in the northern Taiwan.15The SOC reached maxima in summer in T aichung,Pingtung,Penghu,and Hualien (the only exception of Tainan is unclear yet).It is noteworthy that the oxidant exhibited summer minima at the5stations.The strong convection in summertime can strengthen the dispersion and,consequently,reduce the mixing ratios of ozone as well as the otherair pollutants,including SOA.Thus,the summer maxima of SOA indicate that the sea-20sonal enhancement of the production of SOA in summertime overcame the dispersion effects.Considering the reduced oxidant levels,the enhancement of SOA production was most likely a result of increases in the precursor emissions.In this context,the high SOA production in summertime was attributed mostly to the increases in the emissionsof biogenic VOCs,which also show strong seasonality with summer maxima.Besides, 25the SOA production is known being dependent on ambient temperature and relative humidity(Strader et al.,1999;Seinfeld et al.,2001;Russell and Allen,2005).The weather conditions in summer are doubtlessly favorable to the SOA yield and,in turn, to the formation of summer maxima of SOA levels.。