Surface micromachined RF MEMS solenoid inductors and variable capacitors(英文)
RF MEMS
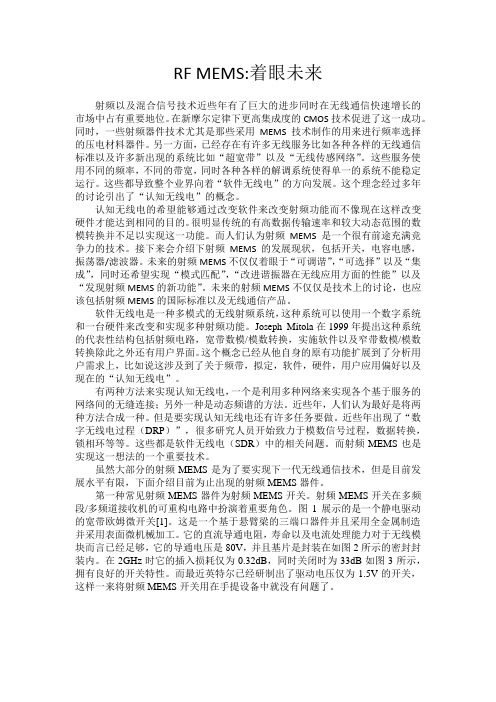
RF MEMS:着眼未来射频以及混合信号技术近些年有了巨大的进步同时在无线通信快速增长的市场中占有重要地位。
在新摩尔定律下更高集成度的CMOS技术促进了这一成功。
同时,一些射频器件技术尤其是那些采用MEMS技术制作的用来进行频率选择的压电材料器件。
另一方面,已经存在有许多无线服务比如各种各样的无线通信标准以及许多新出现的系统比如“超宽带”以及“无线传感网络”。
这些服务使用不同的频率,不同的带宽,同时各种各样的解调系统使得单一的系统不能稳定运行。
这些都导致整个业界向着“软件无线电”的方向发展。
这个理念经过多年的讨论引出了“认知无线电”的概念。
认知无线电的希望能够通过改变软件来改变射频功能而不像现在这样改变硬件才能达到相同的目的。
很明显传统的有高数据传输速率和较大动态范围的数模转换并不足以实现这一功能。
而人们认为射频MEMS是一个很有前途充满竞争力的技术。
接下来会介绍下射频MEMS的发展现状,包括开关,电容电感,振荡器/滤波器。
未来的射频MEMS不仅仅着眼于“可调谐”,“可选择”以及“集成”,同时还希望实现“模式匹配”,“改进谐振器在无线应用方面的性能”以及“发现射频MEMS的新功能”。
未来的射频MEMS不仅仅是技术上的讨论,也应该包括射频MEMS的国际标准以及无线通信产品。
软件无线电是一种多模式的无线射频系统,这种系统可以使用一个数字系统和一台硬件来改变和实现多种射频功能。
Joseph Mitola在1999年提出这种系统的代表性结构包括射频电路,宽带数模/模数转换,实施软件以及窄带数模/模数转换除此之外还有用户界面。
这个概念已经从他自身的原有功能扩展到了分析用户需求上,比如说这涉及到了关于频带,拟定,软件,硬件,用户应用偏好以及现在的“认知无线电”。
有两种方法来实现认知无线电,一个是利用多种网络来实现各个基于服务的网络间的无缝连接;另外一种是动态频谱的方法。
近些年,人们认为最好是将两种方法合成一种。
MEMS致动器原理
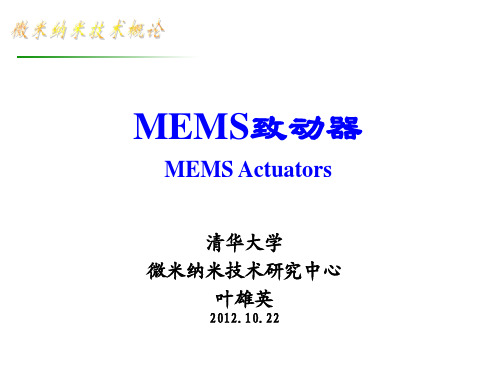
Advantage
Large force Large displacement Low driving voltage
w = t = g = z0 = 2µm Vr =0V C’= dC/dx
Comb Drive Failure Modes
Example
释放应力
Scratch Drive Actuator (SDA)
SDA operation
1) Applied voltage bends SDA downward 2) When released, SDA returns to original shape 3) Reapplying voltage causes SDA to move a distance ‘dx’
Modes in Quartz
压电致动方式
压电致动的主要形式
块状压电晶体:结构紧凑,变形小,频率高; 压电堆:位移较大,致动力很大,轴向尺寸大,对 装配的要求高,驱动频率较高; 压电薄膜:利于系统集成,致动力和位移小; 双层压电梁:驱动力和变形大,频率低。
压电基本方程:
柔度
E 11 1
0.4 mm Mechanical Stop
Mirror
Principle and Features
William Tang, Ph.D. EECS Dept., 1990
Formulae
电场能量: 静电力: C
Energy must include capacitance between the stator and the rotor and the underlying ground plane, which is typically connected at the rotor voltage.
13_-_surface_micromachining
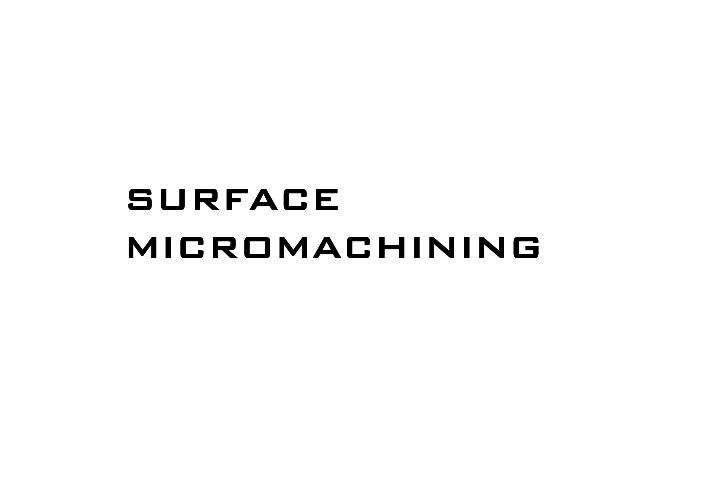
SURFACE MICROMACHININGSURFACE MICROMACHINING•Uses planar technologies to realize microstructures(thin film deposition on the bulk and selective etching)•More complex technology,but also more versatile with respect to bulk micromachining•Concepts of STRUCTURAL layer and SACRIFICIAL layer•Usually allows for better resolutions(and better surface exploitation) •Thickness of devices and cavities are limited(of the order of the micron): NO high structures NOR big masses!!!•STICTION problemSurface Micromachining Substrate (Silicon Wafer)Deposited Thin Film (~µm)Remove PR MASK P h o t o l i t h o g r a p h yEx p osu r eD e v elopEtch FilmSurface MicromachiningDeposit Structural FilmPattern & Etch Structural Film Microbridge MicrocantileverAnchor Selectively Remove First Film (Sacrificial Layer)and signal conditioning circuitry.Examples of microstructures with Surface MicromachiningMICRO GEARS & HINGESFROM SANDIA NATIONALLABS, USABasis for the adhesion of the center pivotSacrificial layer for rotor releaseRotor and statorSacrificial layer to separate rotor from the central pivotCentral pivotElectrical contacts Sacrificial oxide etch for releaseSurface Surface Micromachined Micromachined Inductor•Air bridge can be formed using sacrificial etching.Typical Device Realized by SurfaceMicromachining•Etch holes are required to reduce the time for removing sacrificial layer underneath large-area structures.Criteria for Selecting Materials andEtching Solutions•Selectivity–etch rate on sacrificial layer/etch rate on structural layer must be high •Etch rate–rapid etching rate on sacrificial layer to reduce etching time •Deposition temperature–in certain applications, it is required that the overall processing temperature be low (e.g. integration with CMOS, integration with biological materials)•Intrinsic stress of structural layer–to remain flat after release, the structural layer must have low stress •Surface smoothness–important for optical applications•Long term stabilityOther Structural or SacrificialMaterials•Structural layers–evaporated and sputtered metals such as Gold, Copper–electroplated metal (such as NiFe)–plastic material (CVD plastic)–silicon (such as epitaxy silicon or top silicon in SOI wafer)•Sacrificial layers–Photoresist,polyimide, and other organic materials–Copper•copper can be electroplated or evaporated, and is relativelyinexpensive.–Oxide by plasma enhanced chemical vapor deposition (PECVD)•PECVD is done at lower temperature, with lower quality. It isgenerally undoped.–Thermally grown oxide•relatively low etch rate in HF.–Silicon or polysilicon•removed by gas phase silicon etchingEvaporation DryingLiquid-gas transition is harsh on MEMSTemperature P r e s s u r e BoilingPoint1atmRelease Stiction•Capillary forces of drying rinse solution pull down microstructures–Van der Waals forces are responsible for thestiction of hydrophobic surfaces–Hydrogen bonding is the dominant adhesionmechanism for hydrophilic surfaces–will devices be released after drying?•Low surface-tension liquids are best –Methanol (1st possible solution)Stiction Reduction Strategies•Reduce adhesion area–dimples or bump–low surface-energy coatings•Integrate supporting microstructures –increase tolerance of capillary forces(microfuses,photoresist sacrificial supportinglayer, …)•Sublimation drying•Supercritical drying•Vapor phase etchingStiction ReductionReduction ––Dimples or Bumps Array•Reduces contact area•Stiction is limited also duringoperative life (it may occurs forexample for humiditycondensation)Reduction ––Stiction ReductionLow Surface--Energy MaterialsLow Surface•Adoption of thin fluorinated coating to obtain an hydrophobic surface(teflon-like materials or SAM)Temporary Supporting Structures --Temporary Supporting StructuresMicrofuses•Microfuses provide mechanical support to prevent stiction•Current pulses blow fuses to release devicesSublimation DryingNo direct change from the liquid to the gaseous phase T-butyl alcohol –freezes at 26 ºCP-dichlorobenzene –freezes at 56 ºCTemperatureP r e s s u r e 1atm FreezingPointSublimation Drying Setup•Chip-scale refrigeration (temperature control with Peltier cell to implement slow variations of temperature avoiding microcracking of the microstructures)•Desiccation prevents moisture issues (to avoid acqueos vapor condensation and consequently stiction)Material T c (ºC)P c (atm)P c (psi)Water 3742183204Methanol 240801155CO 231731073TemperatureP r e s s u r e1atmP c T cThe liquid phase is removed with CO 2at a temperature and pressure higher than the critical point (where surface tension is almost zero)•Reaches very high pressures (> 70atm)–very strong chambers areneeded•Automated systems areavailableVapor Phase EtchingAnhydrous HF vapor used to avoid liquid-gas transitionOrDry Etching (plasma etching or O 2RIE with V BIAS = 0for photoresistsacrificial etching),but not easy underdiffusionTemperatureP r e s s u r e< 1atmIn In--Use Stiction•After a successful release–no release stiction•Physical contact during use–electrostatic pull down•Reduce stiction with low surface-energy coatings (FC, SAM, roughening, dimples, bumps,…)。
电子科大微机电系统(MEMS)概论课件 第一章
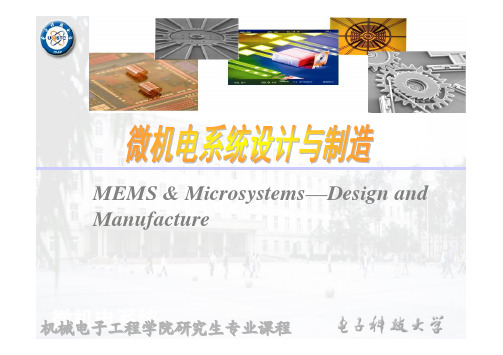
力学
流体
声学
传热学 光学
MEMS
电/磁学
生物学
化学
量子力学
一、MEMS的形成与发展
1、MEMS的形成基础
与机械电子学的关系
• 基本组成相同 • 不是简单的提升
定子
转子
扭矩传 递齿轮
LIGA工艺生成的微马达
MEMS系统框图
MEMS的组成要素:微型传感器、微执行器、信号处理控制电路、通 信系统、微电子电源
Electron Devices ED-35
724–30
旋转式静电微电机 Rotary Electrostatic Micromotor
Fan Long-Shen, Tai Yu-Chong and
Muller R S 1989 IC-processed
Aelcetcutarotostrasti2c0m4i1c–r7omotors Sensors
阻量 =势能变化 / 速度、电流或流量的变化 容量 =质量或位移变化/ 势能变化 惯量 =势能变化/ 流量(速度或电流)每秒的变化
三、MEMS的制造方法概述
MEMS与IC工艺追求不同 • 从二维到“假三维” 、 “真三维” • 以IC平台发展起来为主,非IC工艺日渐丰富
三、MEMS的制造方法概述
5)IC器件主要是电信号,而MEMS器件有机械、光、电、多种信号;
6)IC主要是表面加工工艺,而MEMS有多种加工工艺;
7)IC主要是半导体材料,而MEMS有多种加工材料。
机械学 机械功能
(输入/输出 )
•“系统Systems”——结构,设备,系统水平
MEMS——21世纪微型化的前沿技术
2、MEMS的特点
微型机械 VS 普通机械
mems surface micromachined accelerometer
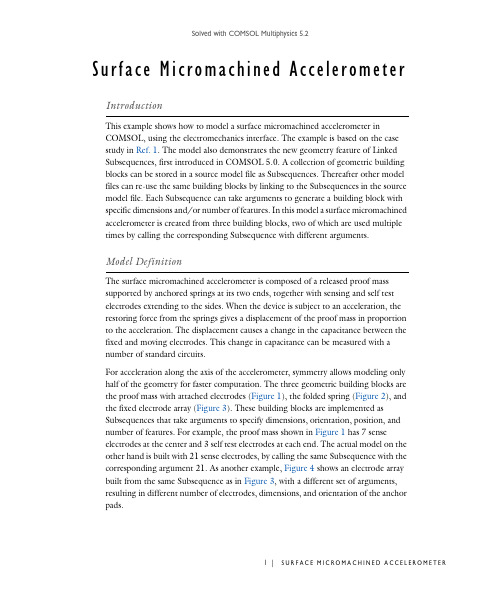
Surface Micromachined Accelerometer IntroductionThis example shows how to model a surface micromachined accelerometer inCOMSOL, using the electromechanics interface. The example is based on the case study in Ref. 1. The model also demonstrates the new geometry feature of Linked Subsequences, first introduced in COMSOL 5.0. A collection of geometric building blocks can be stored in a source model file as Subsequences. Thereafter other model files can re-use the same building blocks by linking to the Subsequences in the source model file. Each Subsequence can take arguments to generate a building block with specific dimensions and/or number of features. In this model a surface micromachined accelerometer is created from three building blocks, two of which are used multiple times by calling the corresponding Subsequence with different arguments.Model DefinitionThe surface micromachined accelerometer is composed of a released proof mass supported by anchored springs at its two ends, together with sensing and self test electrodes extending to the sides. When the device is subject to an acceleration, the restoring force from the springs gives a displacement of the proof mass in proportion to the acceleration. The displacement causes a change in the capacitance between the fixed and moving electrodes. This change in capacitance can be measured with a number of standard circuits.For acceleration along the axis of the accelerometer, symmetry allows modeling only half of the geometry for faster computation. The three geometric building blocks are the proof mass with attached electrodes (Figure 1), the folded spring (Figure 2), and the fixed electrode array (Figure 3). These building blocks are implemented asSubsequences that take arguments to specify dimensions, orientation, position, and number of features. For example, the proof mass shown in Figure 1 has 7 senseelectrodes at the center and 3 self test electrodes at each end. The actual model on the other hand is built with 21 sense electrodes, by calling the same Subsequence with the corresponding argument 21. As another example, Figure 4 shows an electrode array built from the same Subsequence as in Figure 3, with a different set of arguments, resulting in different number of electrodes, dimensions, and orientation of the anchor pads.micrometers.Figure 2: Building block for the folded spring. Grid scales are in micrometers.Figure 3: Building block for the fixed electrode array. Grid scales are in micrometers.Figure 4: Example of an electrode array built from the same Subsequence as in Figure 3, with a different set of arguments. Grid scales are in micrometers.The geometry sequence begins by calling the proof mass Subsequence, followed by calling the folded spring Subsequence twice to attach a spring at each end of the proof mass. Subsequently 6 calls to the fixed electrode array Subsequence are made to construct the sense and self test fixed electrodes.Each Subsequence call contributes to a domain and a boundary selection. This allows easy assignment of domain physics and boundary conditions in the physics interface. The model uses polysilicon for the building material and includes a rectangular air domain surrounding the polysilicon. The Electromechanics interface models the electric field within the deforming gaps between the electrodes, and applies the appropriate electrostatic forces to the solids, which creates a corresponding structural deformation. The narrowing electrostatics domain results in nonlinear geometrical effects which are included in the Electromechanics interface by default.The entire polysilicon solid is subject to an acceleration using the Body Load domain physics feature. The (mechanically) fixed electrodes are set at constant potentials, and the proof mass (and its attached electrodes) is at a floating potential whose value will be determined by the position-dependent capacitance (and the applied voltages on the fixed sense electrodes).Results and DiscussionThe first study illustrates the normal operation of the accelerometer by sweeping the applied acceleration from -50 to +50 g and computing the resulting displacement of the proof mass. Figure 5 shows the displacement of the polysilicon domains when the applied acceleration is 50 g. The proof mass (and the attached moving electrodes)moves by about 0.07 micrometer. The anchored spring bases and the fixed electrodeshave very little movement. The folded springs have varying displacement along its length as expected.Figure 5: Displacement of the polysilicon domains when the applied acceleration is 50 g.The proof mass moves by about 0.07 micrometer. The anchored spring bases and the fixed electrodes have very little movement. The springs have varying displacement along its length as expected.Figure 6: Displacement vs acceleration.Figure 6 shows the linear relationship between the displacement and the applied acceleration. The displacement is measured via the capacitive coupling between the moving and the fixed sense electrodes. In the real device, during normal operation, the proof mass with its attached moving electrodes is floating at a potential close to one half of the supply voltage, and a high frequency square wave swinging between zero and the full supply voltage is applied with opposite phase to the fixed sense electrodes on each side of the moving electrodes. The fixed self test electrodes are biased at one half of the supply voltage. When the proof mass moves as a result of the acceleration, an alternating voltage in proportion to the displacement is induced due to the capacitive coupling between the fixed and moving electrodes. This arrangement nulls the average electrostatic force between the fixed and moving sense electrodes, and facilitates easier signal processing in the attached circuitry. In this example the stationary part of the square wave is modeled using a stationary study, so that the problem solves relatively quickly. The bias is shifted to zero for convenience, and the amplitude of the square wave is divided by an artificial factor of 1000 to reduce the electrostatic force between the fixed and moving sense electrodes (in practice the time average of the force will be zero due to the high frequency excitation). For a 5 V supply in the physical device, this corresponds to applying a +/-2.5 mV on the right-side and left-side fixed sense electrodes in the model. In post processing the artificial factor of1000 is multiplied back to the sensed voltage of the proof mass. Figure 7 shows the linear relationship between the sense voltage and the acceleration. This signal is fed into an amplifier that in the real device was built on the same substrate as theFigure 7: Sensed voltage vs. applied acceleration.The accelerometer in the model was designed with self test electrodes that could be employed to calibrate the device in the factory. The second study illustrates the self testing by applying a bias of 2 V on the fixed self test electrodes, which are at the side of the moving electrodes attached to the proof mass. The electric field between the fixed and the moving electrodes exerts an electrostatic force that causes the proof mass to move. Figure 8 shows the displacement of the polysilicon domains when 0 V is applied to the fixed self test electrodes on the left-hand side of the moving electrodes attached to the proof mass, and 2 V to those on the right-hand side. The proof mass moves by about 0.02 μm, which is large enough in magnitude for the self test purpose (compared to the 0.07 μm of full range displacement shown in Figure 5 and Figure 6).Figure 8: Displacement of the polysilicon domains when 0 V is applied to the fixed self test electrodes on the left-hand side of the moving electrodes attached to the proof mass, and 2 V to those on the right-hand side. The proof mass moved by about 0.02 μm, which is large enough in magnitude for the self test purpose (compared to the 0.07 μm of full range displacement shown in Figure 5 and Figure 6).Figure 9 compares the displacement obtained from applying the self test voltage to each side of the fixed electrodes. The displacement values have the same magnitudewith opposite signs, as expected from symmetry.Figure 9: Displacement vs. applied self test voltage.References1. S.D. Senturia, Microsystem Design 5th ed., Kluwer Academic Publishers, pp. 513–525, 2003.Application Library path: MEMS_Module/Sensors/surface_micromachined_accelerometerModeling InstructionsLoad the geometry file.R O O T1From the File menu, choose Open.2Browse to the application’s Application Library folder and double-click the file surface_micromachined_accelerometer_geom_sequence.mph.The model geometry has been set up using parts in a linked file. It is easier to visualize with wireframe rendering.C O M P O N E N T1(C O M P1)1Click the Wireframe Rendering button on the Graphics toolbar.2Click the Zoom Extents button on the Graphics toolbar.A D D M A T E R I A L1On the Home toolbar, click Add Material to open the Add Material window.2Go to the Add Material window.3In the tree, select Built-In>Air.4Click Add to Component 1.A D D M A T E R I A L1Go to the Add Material window.2In the tree, select MEMS>Semiconductors>Si - Polycrystalline Silicon.3Click Add to Component 1.M A T E R I A L SSi - Polycrystalline Silicon (mat2)1In the Model Builder window, under Component 1 (comp1)>Materials click Si - Polycrystalline Silicon (mat2).2In the Settings window for Material, locate the Geometric Entity Selection section. 3From the Selection list, choose Polysilicon.4On the Home toolbar, click Add Material to close the Add Material window.E L E C T R O M E C H A N I C S(E M I)Linear Elastic Material 11On the Physics toolbar, click Domains and choose Linear Elastic Material.2In the Settings window for Linear Elastic Material, locate the Domain Selection section.3From the Selection list, choose Polysilicon.4In the Model Builder window, collapse the Electromechanics (emi) node.Body Load 11On the Physics toolbar, click Domains and choose Body Load.2In the Settings window for Body Load, locate the Force section.3Specify the F V vector asacceleration*emi.rho*g_const x0y0z4Locate the Domain Selection section. From the Selection list, choose Polysilicon.Symmetry 11On the Physics toolbar, click Boundaries and choose Symmetry.2In the Settings window for Symmetry, locate the Boundary Selection section.3From the Selection list, choose Symmetry plane.Fixed Constraint 11On the Physics toolbar, click Boundaries and choose Fixed Constraint.2In the Settings window for Fixed Constraint, locate the Boundary Selection section.3From the Selection list, choose Anchor plane.Ground 11On the Physics toolbar, click Boundaries and choose Ground.2Select Boundary 45 only.Termin al 11On the Physics toolbar, click Boundaries and choose Terminal.2In the Settings window for Terminal, locate the Terminal section.3From the Terminal type list, choose Voltage.4In the V0 text field, type -2.5[mV].5Click the Zoom Extents button on the Graphics toolbar.6Locate the Boundary Selection section. From the Selection list, choose Sense left boundaries.7Right-click Terminal 1 and choose Rename.8In the Rename Terminal dialog box, type Sense Terminal L in the New label text field.9Click OK.Termin al 21On the Physics toolbar, click Boundaries and choose Terminal.2In the Settings window for Terminal, locate the Boundary Selection section.3From the Selection list, choose Sense right boundaries.4Locate the Terminal section. From the Terminal type list, choose Voltage.5In the V0 text field, type 2.5[mV].6Right-click Terminal 2 and choose Rename.7In the Rename Terminal dialog box, type Sense Terminal R in the New label text field.8Click OK.Floating Potential 11On the Physics toolbar, click Boundaries and choose Floating Potential.2In the Settings window for Floating Potential, locate the Boundary Selection section.3From the Selection list, choose Proof mass boundaries.Termin al 31On the Physics toolbar, click Boundaries and choose Terminal.2In the Settings window for Terminal, locate the Terminal section.3From the Terminal type list, choose Voltage.4In the V0 text field, type VtestL.5Right-click Terminal 3 and choose Rename.6In the Rename Terminal dialog box, type Self Test Terminal L in the New label text field.7Click OK.8In the Settings window for Terminal, locate the Boundary Selection section.9From the Selection list, choose Self test left boundaries.Termin al 41On the Physics toolbar, click Boundaries and choose Terminal.2In the Settings window for Terminal, locate the Boundary Selection section.3From the Selection list, choose Self test right boundaries.4Locate the Terminal section. From the Terminal type list, choose Voltage.5In the V0 text field, type VtestR.6Right-click Terminal 4 and choose Rename.7In the Rename Terminal dialog box, type Self Test Terminal R in the New label text field.8Click OK.M E S H1Free Triangular 11In the Model Builder window, under Component 1 (comp1) right-click Mesh 1 and choose More Operations>Free Triangular.2In the Settings window for Free Triangular, locate the Boundary Selection section.3From the Selection list, choose Meshing plane.4Click the Build Selected button.Swept 1In the Model Builder window, right-click Mesh 1 and choose Swept.Distribution 11In the Model Builder window, under Component 1 (comp1)>Mesh 1 right-click Swept1 and choose Distribution.2In the Settings window for Distribution, locate the Domain Selection section.3Click Clear Selection.4Select Domain 3 only.5Click the Build All button.S T U D Y11In the Model Builder window, right-click Study 1 and choose Rename.2In the Rename Study dialog box, type Study 1: Normal Operation in the New label text field.3Click OK.S T U D Y1:N O R M A L O P E R A T I O NStep 1: Stationary1In the Model Builder window, expand the Study 1: Normal Operation node, then click Step 1: Stationary.2In the Settings window for Stationary, click to expand the Study extensions section.3Locate the Study Extensions section. Select the Auxiliary sweep check box.4Click Add.5Click Range.6In the Range dialog box, type -50 in the Start text field.7In the Step text field, type 25.8In the Stop text field, type 50.9Click Add.10On the Home toolbar, click Compute.R E S U L T S1D Plot Group 3On the Home toolbar, click Add Plot Group and choose 1D Plot Group.Point Graph 1On the 1D Plot Group 3 toolbar, click Point Graph.1D Plot Group 31In the Settings window for Point Graph, locate the Selection section.2Select the Active toggle button.3Select Point 65 only.4Click Replace Expression in the upper-right corner of the y-axis data section. From the menu, choose Component 1>Electromechanics (SolidMechanics)>Displacement>Displacement field (Material)>u - Displacement field, X component.5In the Model Builder window, right-click 1D Plot Group 3 and choose Rename.6In the Rename 1D Plot Group dialog box, type Displacement vs. Acceleration in the New label text field.7Click OK.8In the Settings window for 1D Plot Group, locate the Plot Settings section.9Select the x-axis label check box.10In the associated text field, type Acceleration (g).11On the Displacement vs. Acceleration toolbar, click Plot.1D Plot Group 4On the Home toolbar, click Add Plot Group and choose 1D Plot Group.Global 1On the 1D Plot Group 4 toolbar, click Global.1D Plot Group 41In the Settings window for Global, locate the y-Axis Data section.2In the table, enter the following settings:Expression Unit Descriptionemi.V0_fp1*1000mV Raw sense voltage3Click to expand the Legends section. Clear the Show legends check box.4On the 1D Plot Group 4 toolbar, click Plot.5In the Model Builder window, right-click 1D Plot Group 4 and choose Rename.6In the Rename 1D Plot Group dialog box, type Sense V vs. Acceleration in the New label text field.7Click OK.R O O TOn the Home toolbar, click Windows and choose Add Study.A D D S T U D Y1Go to the Add Study window.2Find the Studies subsection. In the Select study tree, select Preset Studies>Stationary. 3Click Add Study.S T U D Y2Step 1: Stationary1On the Home toolbar, click Add Study to close the Add Study window.2In the Model Builder window, under Study 2 click Step 1: Stationary.3In the Settings window for Stationary, locate the Study Extensions section.4Select the Auxiliary sweep check box.5Click Add.6In the table, enter the following settings:Parameter name Parameter value list Parameter unit VtestL 2 07Click Add.8In the table, enter the following settings:Parameter name Parameter value list Parameter unit VtestR0 29From the Run continuation for list, choose No parameter.10In the Model Builder window, right-click Study 2 and choose Rename.11In the Rename Study dialog box, type Study 2: Self Test in the New label text field.12Click OK.13On the Home toolbar, click Compute.R E S U L T SDisplacement (emi) 1Click the Zoom Extents button on the Graphics toolbar.Potential (emi) 11In the Model Builder window, expand the Potential (emi) 1 node, then click Slice 1. 2In the Settings window for Slice, locate the Plane Data section.3In the Planes text field, type 8.4On the Potential (emi) 1 toolbar, click Plot.1D Plot Group 71On the Home toolbar, click Add Plot Group and choose 1D Plot Group.2In the Settings window for 1D Plot Group, locate the Data section.3From the Data set list, choose Study 2: Self Test/Solution 2 (sol2).Point Graph 1On the 1D Plot Group 7 toolbar, click Point Graph.1D Plot Group 71Select Point 65 only.2In the Settings window for Point Graph, click Replace Expression in the upper-right corner of the y-axis data section. From the menu, choose Component1>Electromechanics (Solid Mechanics)>Displacement>Displacement field (Material)>u -Displacement field, X component.3Locate the x-Axis Data section. From the Parameter list, choose Expression.4In the Expression text field, type VtestR.5On the 1D Plot Group 7 toolbar, click Plot.6In the Model Builder window, right-click 1D Plot Group 7 and choose Rename.7In the Rename 1D Plot Group dialog box, type Displacement vs. Self Test V in the New label text field.8Click OK.。
微机械陀螺仪
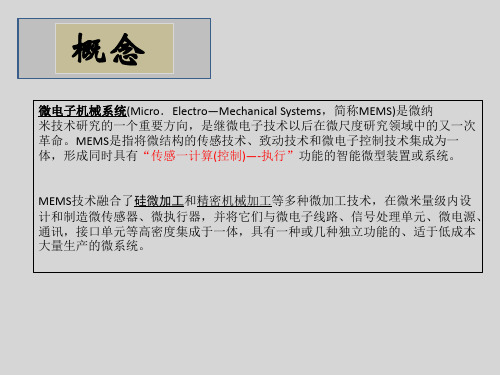
微电子机械系统(Micro.Electro—Mechanical Systems,简称MEMS)是微纳 米技术研究的一个重要方向,是继微电子技术以后在微尺度研究领域中的又一次 革命。MEMS是指将微结构的传感技术、致动技术和微电子控制技术集成为一 体,形成同时具有“传感一计算(控制)—-执行”功能的智能微型装置或系统。
2023/12/29
其中硅基微加工技术,又包括体硅微制造(Bulk Micromaching)、表面微加工(Surface Micromaching)。
பைடு நூலகம்
LIGA技术
LIGA是德文Lithografle,Galvanofomung,Abformun93个词,即 光刻、电铸及进塑的缩写。
LIGA技术是种基于x射线光刻技术的三维微结构加工技术,主要包括x 光深度同步辐射光刻,电铸制模和注模复制3个工艺步骤,与其它立体 微加工技术相比.LIGA工艺具有如下特点:
在表面微机械加工工艺中,机械加工层越多可制造的微型机械越复杂,功能 越强大,但是微型元件的布局问题、平面化问题和减小残余应力问题也更难 解决。
发展
今后微机械陀螺仪的主要研究内容为:
①进一步研究微机械振动陀螺仪的结构及电路的优化设计,实现惯性级高性能的要 求,其中高灵敏度、低噪声、低漂移和大动态范围的测试电路是提高微机械陀螺仪 的关键所在; ②研究微机械振动陀螺仪的体加工和表面加工技术; ③研究电路的模块设计及加工技术: ④完善微机械振动陀螺系统的封装及测试标定。
比较
所有研究开发的硅微机械陀螺仪都是利用振动质量块在柱物体带动旋转时产生的哥氏 效应来测量物体旋转的角速度。硅微机械陀螺仪的结构形式要包括两部分.驱动部分 和敏感部分。目前,硅微机械陀螺仪常采用的驱动方式有静电驱动、电磁驱动等,检 测方式般为电容检测、电磁检测以及压电检测。
MEMS receiver,filter,cmos mems
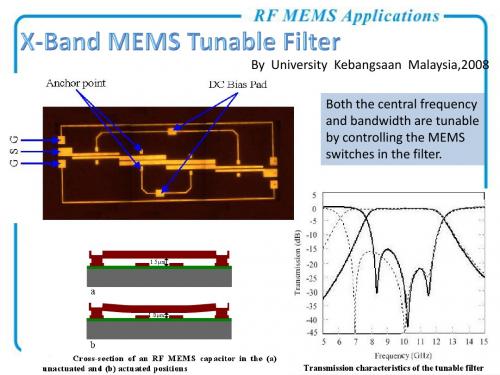
MEMS-Enabled Dual-band 1.8& 5-6GHz Receiver RF Front-end
A Programmable Complex FIR Filter with Integrated MEMS Filter for Front-end Charge Sampling Receiver (By STMicroelectronics,2006)
Oscillator Phase Noise @2.2GHz
Micromachined Silicon Rectangular Waveguide at 400 GHz (Georgia Tech,2006)
The silicon waveguide has an average loss of 0.086 dB/mm for a range of 350–460 GHz. The waveguides are formed using microfabrication techniques and demonstrate a successful first step towards the use of silicon waveguides as a viable option for THz systems.
By University Kebangsaan Malaysia,2008
Both the central frequency and bandwidth are tunable by controlling the MEMS switches in the filter.
4-Bit 10-14GHz RF MEMS Tunable Filter
There are several successful examples of VCO by using post-foundry CMOS micro-machined high Q inductor or tunable capacitor.
SURFACE MICROMACHINED MICRONEEDLES
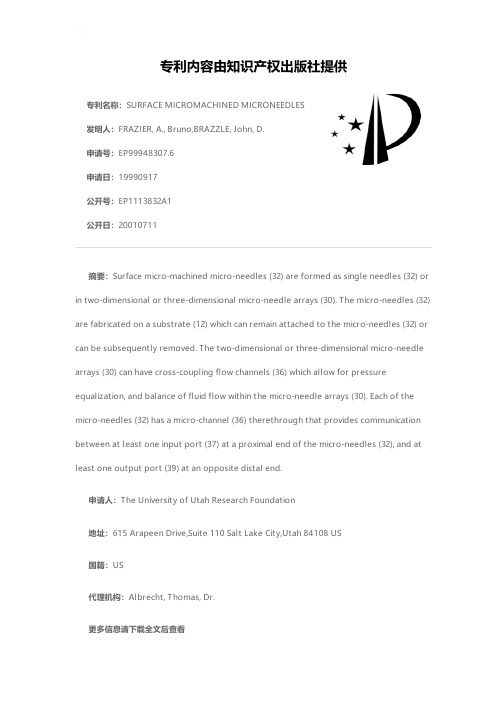
专利名称:SURFACE MICROMACHINED MICRONEEDLES 发明人:FRAZIER, A., Bruno,BRAZZLE, John, D.申请号:EP99948307.6申请日:19990917公开号:EP1113832A1公开日:20010711专利内容由知识产权出版社提供摘要:Surface micro-machined micro-needles (32) are formed as single needles (32) or in two-dimensional or three-dimensional micro-needle arrays (30). The micro-needles (32) are fabricated on a substrate (12) which can remain attached to the micro-needles (32) or can be subsequently removed. The two-dimensional or three-dimensional micro-needle arrays (30) can have cross-coupling flow channels (36) which allow for pressure equalization, and balance of fluid flow within the micro-needle arrays (30). Each of the micro-needles (32) has a micro-channel (36) therethrough that provides communication between at least one input port (37) at a proximal end of the micro-needles (32), and at least one output port (39) at an opposite distal end.申请人:The University of Utah Research Foundation地址:615 Arapeen Drive,Suite 110 Salt Lake City,Utah 84108 US国籍:US代理机构:Albrecht, Thomas, Dr.更多信息请下载全文后查看。
Surface micromachined millimeter-scale RF system a
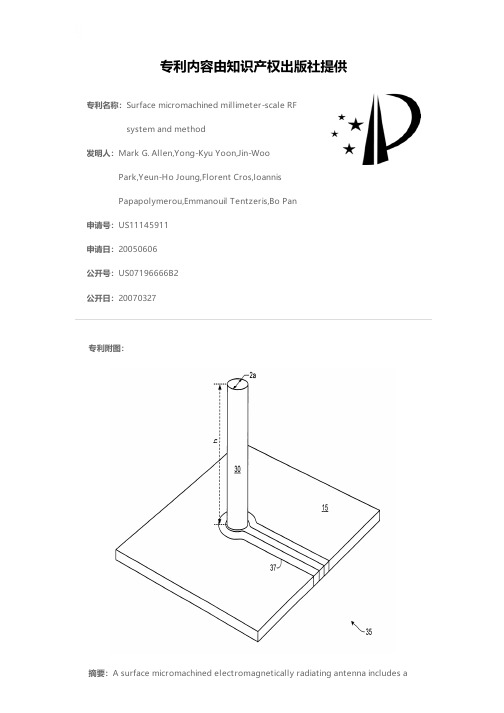
专利名称:Surface micromachined millimeter-scale RFsystem and method发明人:Mark G. Allen,Yong-Kyu Yoon,Jin-WooPark,Yeun-Ho Joung,Florent Cros,IoannisPapapolymerou,Emmanouil Tentzeris,Bo Pan申请号:US11145911申请日:20050606公开号:US07196666B2公开日:20070327专利内容由知识产权出版社提供专利附图:摘要:A surface micromachined electromagnetically radiating antenna includes acoplanar waveguide on a ground plane coated substrate having a conductor path. The conductor path is coupled to a monopole conductor, which has a generally-cylindrical backbone erected vertically from the substrate and a metal layer deposited on the backbone at a predetermined thickness. The antenna may be fabricated by depositing an epoxy on the ground plane coated substrate to a predetermined depth and according to a pattern. The epoxy is exposed to an ultraviolet source that develops one or more columns according to the pattern. A seed layer of metal may be formed on the developed column. A conductive metal is electrodeposited over the column surface to produce the monopole antenna. Other antenna may be created by adding monopoles and/or conductive metal patches and/or strips that are positioned atop the monopoles and elevated from the substrate.申请人:Mark G. Allen,Yong-Kyu Yoon,Jin-Woo Park,Yeun-Ho Joung,FlorentCros,Ioannis Papapolymerou,Emmanouil Tentzeris,Bo Pan地址:Atlanta GA US,Smyrna GA US,Suwanee GA US,Suwanee GA US,Decatur GAUS,Decatur GA US,Atlanta GA US,Atlanta GA US国籍:US,US,US,US,US,US,US,US代理机构:Thomas, Kayden, Horstemeyer & Risley LLC更多信息请下载全文后查看。
机电工程与自动化学院微机电系统(MEMS)

微型动力系统
微型动力系统以电、热、动能或机械能的 输出为目的,以毫米到厘米级尺寸,产生瓦到 十瓦级的功率。 MIT从1996年开始了微型涡软发动机的研 究,该微型涡轮发动机利用MEMS加工技术制 作,主要包括一个空气压缩机、涡软机、燃烧 室、燃料控制系统(包括泵、阀、传感器等)、 以及电启动马达/发电机。该校已在硅片上制作 出涡轮机模型。其目标是1cm直径的发动机产 生10~20W的电力或0.05~0.01N的推力,最终 达到100W。
微机电系统研究得到了美国、日本、欧洲等各 国政府机构、企业界、高等学校与研究机构的高度 重视。源源不断涌现的MEMS器件和系统不但突破 了某些传统加工技术的瓶颈,实现了技术的进步, 甚至带来了观念的革命和产业结构的更新。其中以 美国的表现最为突出。 从八十年代以来,美国政府机构把MEMS作为 科技发展的三大重点之一,先后得到了NASA、 DARPA、国家科学基金会等数亿美元的资助。1994 年发布的《美国国防部技术计划》报告也将微机电 系统列为关键技术项目。
MEMS加工技术
MEMS加工技术主要有从半导体加工工艺中发 展起来的硅表面工艺和体硅工艺。八十年代中期以 后利用X射线光刻、电铸、及注塑的LIGA(德文 Lithograph Galvanformung und Abformug简写)技术 诞生,形成了MEMS加工的另一个体系。MEMS的加 工技术可包括硅表面加工和体加工的硅微细加工、 LIGA加工和利用紫外光刻的准LIGA加工、微细电火 花加工(EDM)、超声波加工、等离子体加工、激 光加工、离子束加工、电子束加工、立体光刻成形 等。MEMS的封装技术也很重要。传统的精密机械加 工技术在制造微小型机械方面仍有很大潜力。
4)集成化:可以把不同功能、不同敏感方向或 致动方向的多个传感器或执行器集成于一体, 或形成微传感器阵列、微执行器阵列,甚至把 多种功能的器件集成在一起,形成复杂的微系 统。微传感器、微执行器和微电子器件的集成 可制造出可靠性、稳定性很高的MEMS。
伯克利课程MEMS设计IntroductiontoMEMSdesign3

• These properties, combined with silicon’s exceptional mechanical characteristics, and well-developed manufacturing base, make silicon the ideal material for precision sensors
compressive oxide still showing
(lower right)
MEMS Design & Fab
ksjp, 7/01
(100)
(110) (111)
(110)
(111)
(110)
Clever KOH etching of (100)
Clockwise from above: Ternez; Rosengren; Keller
ksjp, 7/01
MEMS Design & Fab
Anisotropic Etching of Silicon
<111>
<100>
54.7 Silicon Substrate
• Anisotropic etches have direction dependent etch rates in crystals • Typically the etch rates are slower perpendicularly to the crystalline
MEMS Design & Fab
ksjp, 7/01
Surface micromachining material systems
【微电子机械系统技术 哈工大】MEMS-Fab3

and then spinning and …..(1991)
• Using an integrated polymer support
structure(1993)
Modular Processes
• CMOS before MEMS
oxide mask layer Si device layer, 20 µm thick buried oxide layer
Si handle wafer
2) Etch the silicon device layer to expose the buried oxide layer.
silicon Thermal oxide
5. MEMS stress anneal
9. Trench opened, MEMS released
Analog Devices BiMEMS Process
• ADXL50 accelerometer • Interleaved MEMS and 4 µm BiMOS fabrication • MEMS-CMOS interconnect by diffused n+ runners • Relatively deep junctions allow for MEMS poly stress anneal • Acceleration to volt transducer • Measurement of ±50 g accelerations
5. Other Tools • Chemical and photochemical milling • Photofabrication • Electrochemical machining • Electrodischarge machining (+ wire
MEMS微系统 复习红宝书(北理)

20.BGA : Ball Grid Array 球状矩阵排列
21.SHM:Structural Health Monitoring 结构健康监测
22.ICT:Information and Communications Technologies 信息与通信技术
23.MtM More than Moore 超越摩尔定律
24.FEA:Finite Element Analysis 有限元分析
25.SEM:Scanning Electron Microscope 扫描电子显微镜
12.ITRS International technology Roadmap for Semiconductor 国际半导体技术规
划
.
27.DARPA :Defence Advanced Research Projects Agency of theDepartment of
成,它们各具不同的能带隙。这些材料可以是 GaAs 之类的化合物,也可以是 Si-Ge 之类的半导体合金。按异质结中两种材料导带和价带的对准情况可以把异 质结分为Ⅰ型异质结和Ⅱ型异质结两种。 12.微加工:以微小切除量获得很高精度的尺寸和形状的加工。 13.引线键合:引线键合(Wire Bonding)是一种使用细金属线,利用热、压力、 超声波能量为使金属引线与基板焊盘紧密焊合,实现芯片与基板间的电气互连和 芯片间的信息互通。 14. 倒装芯片:倒装芯片(Flip chip)是一种无引脚结构,一般含有电路单元。 设 计用于通过适当数量的位于其面上的锡球(导电性粘合剂所覆盖),在电气上和 机械上连接于电路。 15.热声焊:热声焊是一种固态键合技术,为热压结合与超音波结合的混合方法。 它可完成电路片与芯片、腔体之间的电连接。 16.各向异性粘接:用各向异性导电胶(主要使用单一或双重成分的环氧树脂)完 成对电路基板与倒装芯片之间的互连。 17.柔性印刷电路:即 FPC,是以聚脂薄膜或聚酰亚胺为基材制成的一种具有高度 可靠性,绝佳曲挠性的印刷电路。通过在可弯曲的轻薄塑料片上,嵌入电路设计, 使在窄小和有限空间中堆嵌大量精密元件,从而形成可弯曲的挠性电路。 18.高深宽比:垂直于加工表面的高度与其加工表面上所具有的特征尺寸的比值 大。 19. 盲孔: 定义 1.位于印刷线路板的顶层和底层表面,具有一定深度,用于表层线路和下面 的内层线路的连接,孔的深度通常不超过一定的比率(孔径)。
微机电系统的未来(MEMS)外文文献翻译、中英文翻译、外文翻译
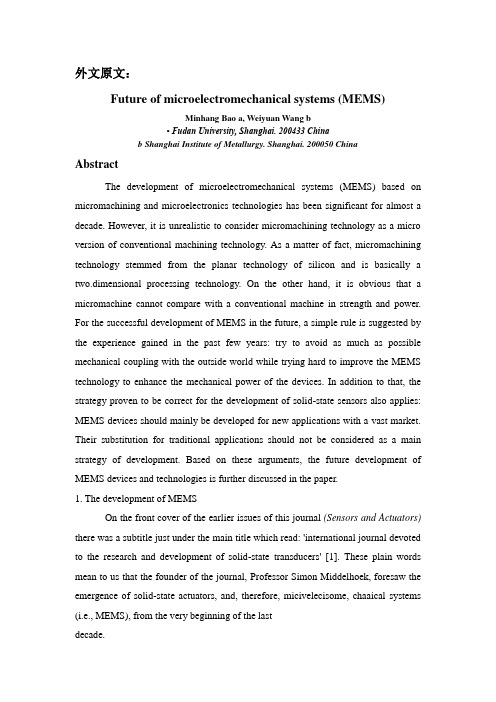
外文原文:Future of microelectromechanical systems (MEMS)Minhang Bao a, Weiyuan Wang b• Fudan University, Shanghai. 200433 Chinab Shanghai Institute of Metallurgy. Shanghai. 200050 ChinaAbstractThe development of microelectromechanical systems (MEMS) based on micromachining and microelectronics technologies has been significant for almost a decade. However, it is unrealistic to consider micromachining technology as a micro version of conventional machining technology. As a matter of fact, micromachining technology stemmed from the planar technology of silicon and is basically a two.dimensional processing technology. On the other hand, it is obvious that a micromachine cannot compare with a conventional machine in strength and power. For the successful development of MEMS in the future, a simple rule is suggested by the experience gained in the past few years: try to avoid as much as possible mechanical coupling with the outside world while trying hard to improve the MEMS technology to enhance the mechanical power of the devices. In addition to that, the strategy proven to be correct for the development of solid-state sensors also applies: MEMS devices should mainly be developed for new applications with a vast market. Their substitution for traditional applications should not be considered as a main strategy of development. Based on these arguments, the future development of MEMS devices and technologies is further discussed in the paper.1. The development of MEMSOn the front cover of the earlier issues of this journal (Sensors and Actuators) there was a subtitle just under the main title which read: 'international journal devoted to the research and development of solid-state transducers' [1]. These plain words mean to us that the founder of the journal, Professor Simon Middelhoek, foresaw the emergence of solid-state actuators, and, therefore, micivelecisome, chaaical systems (i.e., MEMS), from the very beginning of the lastdecade.MEMS are integrated systems consisting of microelectronics (IC), microactuators and, in most cases, microsensors. Microelectronics technology has been developing rapidly since 1960 and has been quite mature since the 1970s. Micromachining technology, the technology for mechanical sensors and microactuators, has been developing almost in parallel with microelectronics technology, though the former has lagged far behind in sophistication.At an earlier stage, the development of micromachining was focused on bulk micromachining mainly associated with solid-state pressure transducers. People at the time did not expect too much from the integration of micromechanical structures and microelectronics because bulk-micromachin-ing technology as well as solid-state pressure transducers were relatively difficult to integrate with microelectronics.In 1987, the first movable micromechanical parts were fabricated by surface-micromachining technology [2] and a typical microactuator, the electrostatic micromotor, was successfully operating in the next year [3]. Due to the high compatibility between the surface micromachining and microelectronics technologies, the integration between micromachines and microelectronics led to the birth of MEMS in the following years.As there has been no clear definition of MEMS, in this article we consider a typical MEMS device as:(1) A device that consists of a micromachine and microelectronics, where the micromachines are controlled by microelectronics. Quite often, microsensors are involved in the control system by providing signals to the microelectronics.(2) A device that is fabricated using micromachining technology and an IC process, i.e.. technologies of batch fabrication.(3) A device that is integratedly born, without individual assembly steps for the main parts of the device except for the steps required for packaging.These points mean that a pressure transducer is not considered as a typical MEMS device but as a mechanical sensor as there is no microclectronics control over micromechanical structures. On the other hand, a micromotor is not a typical MEMS device but a typical part ofMEMS D a microactuator.As MEMS are an integration of micromachining technology and microelectronics (IC) technology, they emerged soon after the advent of the micromachine in 1987. The development of MEMS for almost one decade has been significant: various new techniques have been developed, numerous new devices have been designed and fabricated with some of them being commercialized, and research on MEMS has been conducted by almost all major universities and research institutions, enjoying wide support from industries and government agencies. The field has been described as 'growing into a credible, dynamic and popular adolescence from an uncertain child' in less than a decade [4].But every silver lining has a cloud, and this case was no exception. Some problems developed during the rapid development too. The rapid appearance of new micromachines stirred up high expectations from the scientific communities and even public news media. Some tended to believe that the most sophisticated electromechanical system, like a robot, can be copied into a micro version and the micromachine can still do a similar job to its macro counterparts. There have a'oo been outrageous predictions, like microrobots gathering up radioactive particles at toxic sites, and microsubmarines stalking and attacking viruses and cancerous cells while swimming through the bloodsu'eam. The real development can hardly match such a development pattern from the news media. Though micromotors have been designed and fabricated one by one, they run into the common problem of small torque and relatively large friction on the micron scale. People are usually excited simply by the functioning of a micromotor,\ not being able to expect too much in terms ,:~f torque/ power output. It seems impractical to put them into use like a conventional machine. So far no micromachine can replace a conventional machine in any practical application. This situation has given rise to a sceptical cloud over the future of MEMS.However, application is always the final driving force for any emerging technology. Starting at the beginning of this decade, the practicai application of a microactuator has been talked about more and more often by the transducer community and more and more efforts have been made to put microactuators into realapplication.There have been two approaches so far to push forward the application of microactuators. One of the approaches is to make laicroactuators more powerful and stronger. For example, the force produced by a bimetal structure is large enough for many applications, such as to open and close microvalves. This scheme has been quite successful so that the electrocontrolled microvalve has been commercialized. Shape memory alloy (SMA) can also produce a large force and has been considered for similar purposes. For micromotors, electromagnetic motors have been designed and fabricated. The torque can be several orders of magnitude higher than that of an electrostatic micromotor, but there are still quite a lot of problems related to the design and processing.Another approach is to look for some new applications where little force output is required. To do this, direct mechanical coupling between the micromachine and the macro world should be avoided. The interface between the MEMS and the outside world will be through electrical, optical and magnetic signals. This approach has resulted in quite a lot MEMS devices with practical applications; some of them has been commercialized.This successful experience tell us that the future of MEMS is bright if the nature of micromachining technology is respected.2. The nature of micromachining technologyMEMS are the offspring of two modern technologies, the microelectronics and the micromachining technologies. From a technological point of view, there are some affinities between these two technologies. It is well known that microelectronics (IC) technology stemmed from the planar technology of silicon. As a matter of fact, the application of planar processes of silicon to the formation of mechanical structures gave birth to micromachining technology in the 19"/0s. The successful application of planar technology in solid-state pressure transducers promoted the development of micromachining at the early stage.Basically, there are two main categories of micromachining techniques: bulk micromachining and surface micromachining. The techniques are called bulkmicromachining when the bulk material of the substrate (in general silicon) is involved in the process and as surface micromachining if only the deposited (or plated) films on the surface of the substrate are involved in the machining process. Both types of micromachining technologies have the same virtues as microelectronics technology, i.e., high precision and batch fabrication, but they have the same limitations stemming from planar processing technology.First, the structures made by micromechanical technology can be three din~ensional in appearance, but they are two dimensional in essence as they are evolved according to certain rules from planar etching masks. The structures can be made more complicated by repeating the film deposition and masked etching more than once, but the flexibility is still limited by the number of repititions and the processing order starting from the surface of the substrate. Therefore, it is unrealistic to consider micromachining technology as a micro version of conventional machining technology as it has the limitation of the planar process: a basically two-dimensional processing technology not suitable for assembly steps to construct a machine from indiv;.dually processed parts. We cannot expect micromachining technology to be as flexible and versatile as conventional machining in the conventional world. Some selective deposition and etching techniques claimed to have real three-dimensional capability are under. development [5,6], but it is still too early to fores~.e their possible application in practice.Therefore, one simple rule that has to be Ix)me in mind is that all mechanical structures made by conventional mechanical technology cannot he copied in micromechanical versions, and large arrays with simple structure are more suitable for micromachining technology than a single machine with complicated structure.On the other hand, it is obvious that a micromachine can hardly compare with a conventional machine in strength and power. The smaller the structure, the smaller the strength and the power output it can provide. In many cases, micromachines even have difficulties in just running in a conventional environment due to the extra-large friction on the micro scale and the interference of dust, humidity, etc., not to mention on the power output to drive a macromachine.For successful development of MEMS, one more simple rule is suggested: try to avoid as much as possible mechanical power output while trying hard to improve the MEMS technology to enhance the mechanical strength and power of the devices. It is important to respect the nature of a new technology so that its potential can be fully explored. As a matter of fact, there have been many successful experiences in the short history of MEMS by making full use of the advantages and avoiding the disadvantages of micromachining technology.3. The future of MEMS devicesAs mentioned above, for the future development of MEMS technology, two-fold efforts should be made: one is to improve micromachining technologies continuously and the other is to develop appropriate devices for practical applition according to the nature of the MEMS technologies. The latter is an urgent task at present, l'herefore, the first thing we have to address is: what are the appropriate MEMS devices.'?As micromachining technologies have the advantage of high-precision low-cost batch production but the limitations of two-dimensional masking, low strength, low power output and high susceptibility to the interference of many environment factors, such as dust, humidity, etc., the future MEMS devices should be mainly packaged independent subsystems consisting of micromachines" microelectrouics and, in many cases, microsensors. The coupling between the MEMS devices and the outside world would mainly be via electrical, optical, magnetic and other non-contact signals for power supplies, con~xol information, input and output signals. A large array of relatively simple mechanical structures is preferable to complicated mechanical structures.Also, the future MEMS devices should be aimed at new applications with a vast market so that the device can be mass produced to explore fully the advantage of a planar process in low-cost mass production. Here the argument made by Professor Simon Middelhoek [ 7 ] for silicon smart sensors applies to MEMS too: substitution in an old application does not have the potential to create a large enough market. Therefore, it cannot be considered as a main strategy for future MEMS development.As a matter of fact, the above-mentionod approaches have been proven successful in developing MEMS devices in the past and will be adapted for future devel~)pment. A variety of MEMS devices meeting the above-mentioned criteria will be developed in the future, and arc described below.3. MF.MS devices for inertial sensingSilicon accelerometers have been developing rapidly during the last decade and are considered as the next massproduced micromechanical sensor after silicon pressmsensors.The most attractive type of microaccelerometer, the fort.ebalanced accelemmeter, is in fact a MEMS device consisting of a beam-mass mechanical structure, a capacitive sensor for the position of the mass, the signal-processing electronics for the sensor and an electrostatic actuator to apply a feedback force to the seismic mass. A variety of force-balanced microaccelerometers have been developed by now, but so far the most successful one is the fully integrated microaccelerometer, ADXLSO, which was released for production about two yeats ago [8].The mechanical structure of the devices is fabricated by means of polysilicon surface micromachining, and the electronics are fabricated by means of BiCMOS IC technology. Aiming at applications for airbag release control, the operation range of the device is 50g with a single 5 V power supply. The entire microsystem is fabricated on a silicon chip measuring 3 mm× 3 mm and housed in a TO- I00 can. Though the process is considered quite sophisticated and difficult [9], the developer claimed that they can be marketed at a cost under US$15 apiece. Furthermore, an improved version with an operation range as low as 5g or lg has been announced [ 10]. Similar devices based on SIMOX SOI material [ 11 ] and thick epi-polysilicon have also been developed [ 12].Force-balanced microaccelerometers can be considered as one of the most successful MEMS at present. One reason for their success is that the sensing of acceleration by the seismic mass is through non-contact inertial force and the output is an electrical signal so that the whole system can be hermetically sealed in a package to ensure that the performance of the micromechanical structures would not behindered by any environmental interferences. The second reason is that accelerometers can find mass application in a variety of motioncontrol systems. A notable example is for mass applications in airbag control in automobiles. This kind of application spurred significant interest and investment from industry.Another inertial sensing device, the gyroscope, has a similar operation pattern to the accelerometer and can also find wide applications in motion control, including automotive applications such as traction control systems and ride-stabilization systems, consumer electronics applications such as video camera stabilization and model aircraft stabilization, computer applications such as an inertial mouse, robotics applications and, of course, military applications. Therefore, the micromechanical gyroscope has been receiving vigorous development efforts in recent years.As the high-speed rotation parts and bearings in a traditional gyroscope are difficult to miniaturize and batch fabricate by micromechanical technologies to produce low-cost devices, micromechanical gyroscopes are exclusively of vibrating types, including double-gimbals structure [13], cantilever beam structure [ 141, tuning-fork structure [15] and vibrating ring structure [ 16]. Among them the vibrating ring device is the most sophisticated one, which is developed by a LIGA-iike post-circuit process for incorporating highaspect- ratio electroformed metal microstructures with a CMOS circuit for control and readout electronics.Though none of these micromechanical gyroscopes has been commercialized yet, it is quite likely that some form of MEMS gyroscope will be mass produced in the near future.References[1] S. Middelhoek, Sensors and Actuators. ! ( 1981 ) front cover.[2] L.S. Fan, Y.C. Tai and R.S. Muller, Integrated movable micromechanical structure for sensors and actuators, IEEE Trans. Electron Devices. 35 (1988) 724-730.[3l L.S. Fan, Y.C. Tai and R.S. Muller, IC-processed electrostatic micromotor, Proc. 1988 IEEE int. Electron Devices Meeting, San Francisco, CA, USA, !1-14 Dec.. 1988. pp. 666-669.[4] K. Petersen, MIEMS: What lies ahead, Tech. Digest, 8th Int. Conf. Solid.State Sensors and ActuatorslEurosensors IX, Stockholm. Sweden. 25-29.;une, 1995, V ol. 1, pp. 894-897.[5] T.M. Bloomestein and D.J. Ehrlich, Laser deposition and etching of three-dimensional microstructuw~, Tech. Digest, 6th Int. Conf. Solid- State Sensors and Actuators (Transducers '91), San Francisco. CA, USA, 24-28June, 1991, pp. 507-511.[6] H. Westberg, M. Boman, S. Johansson and J. Schweitz, Truly three dimensional structures microfabricated by laser chemical process, Tech. Digest, 6th Int. Conf. Solid.State Sensors and Actuators (Transducers '91), San Francisco, CA, USA, 24-28 June, 1991, pp. 516--519.[7] S. Middelhoek, Seminar at Shanghai Institute of Metallurgy. Chinese Sciences Academy, October 1995.[8] W. Kuehnel and S. Sherman, A surface micromaehined silicon acceleromeler with on-chip detection circuitry, Sensors and Actuators A, 45 (1994) 7-16.[9l T.A. Core, W.K. Tsang and SJ. Sherman. Fabrication technology for an integrated surface micromachined sensor. Solid State Technol., :Oct.) (1993) 39.[ 10] K.H.L. Chart. S.R. Lewis. Y. Zhao, R.T. Howe, S.F. Ban and R.G. Marcheselli, An integrated force-balanced capacitive accelemmeter for Iow-g application. Sensors and Actuators A, 52-54 (1996) 472-476.[ 11 ] L. Zimrnermann et at., Airbag application: a microsystem including a silicon capacitive accelerome~er, CMOS switched capacitor electronics and true self-test capabi.*!ly, sensors and Actuators A. 46-47 (1995) 190-195.[ 12] M. Offenherg, F. I.,arn~r, B. Eisner, H. Munzel and W. Riethmuller, Novel process for monolithic integrated accelerometer, Tech, Digest, 8th int. Conf. Solid-State Sensors andActuators/Eurosensors IX, Stockholm, Sweden, 25-29 June, 1995, Vol. 1, pp. 589-592.[13] P. Greiff, B. Boxenhom, T. King and L. Nilus, Silicon monolithic micromechanical gyroscope, Tech. Digest. 6th Int. Conf. Solid-State Sensors and Actuators (Transducers '91). San Francisco, CA. USA, 24-28 June, 1991. pp. 966-968.[ 14] K. Maenaka and T. Shiozawa, Silicon rate sensor using anisotmpic etching technology, Tech. Digest, 7th Int. Conf. Solid.State Sensors and Actuators (Transducers '93), Yokohama, Japan, 7-10 June, 1993,pp. 642-4545.[ 15 ] J. Soderkvist, Micromachined gyroscopes, Tech. Digest. 7th Int. Conf.Solid-State Sensors and Actuators (Transducers '93), Yokohama,Japan, 7-10June, 1993, pp. 638-641.[ 16 } M.W. Putty and K. Najafi, A micromachined vibrating ring gyroscope,Tech. Digest, Solid-State Sensors and Actuators Workshop. Hilton Head lsland, SC, USA, June 1994, pp. 213-220.[ 17] J.B. Sampsell, The digital micromirror device and its application to project displays, Tech. Digest, 7th Int. Conf. Solid-State Sensors and Actuators (Transducers '93), Yokohama, Japan, 7-10June, 1993, pp.24-27.[ 18] News focus: new flat displays emerge in Japan Electronics Show '95,Nilckei Electronics Asia, (Dec.) ( 1995 ) 28.[19] O. Tabata, R. Asabi, N. Fujitsuka, M. Kimura and S. Sygiyama, Electrostat,.'c driven optical chopper using SOl wafer, Tech. Digest, 7th Int. Conf. Solid-State Sensors and Actuators (Transducers '93),Yokohama, Japan, 7-10 June, 1993, pp. 124--127.[20] E. Obermeier. J. Lin and V. Schkuchting. Design and fabrication of an electrostatistically driven micro-shutter, Tech. Digest, 7th Int. Conf.Solid-State Sensors and Actuators (Transducers '93), Yokohama,Japan. 7-10June, 1993. pp. 132-135.[21 ] S.T. Wilkinson, Y.W. Kim, M.G. Allen and N.M. Jokerst, Integration of thin-film compound semiconductor photonic devices onto micromachined movable platforms, Tech. Digest, 7th Int. Conf. Solid-State Sensors and Actuators (Transducers '93). Yokohama, Japan,7-10June, 1993, pp. 148-151.[221 J. Mohr, M. Kohl and W. Menz, Micro optical switching by electrostatic linear actuators with large displacements, Tech. Digest,7th Int. Conf. Solid-State Sensors and Actuators (Transducers '93),Yokohama, Japan, 7-10June, 1993, pp. 120-123.[23l C.H. Ahn, Y.J. Kim and MG. Allen, A fully integrated micromachined toroidal inductor with a nickel-iron on magnetic core, Tech. Digest,7th Int. Conf. Solid-State Sensors and Actuators fTransducers '93),Yokohama, Japan. 7-10 June, 1993, pp. 70-73.[24] S. Kawahito, Y. Sasaki, M. Ishida and T. Nakamura, A flux-gate magnetic sensor with micromachined solenoids and electroplated pennalloy cores, Tech. Digest, 7th Int. Conf. Solid-State Sensors and Actuators (Transducers '93), Yokohama, Japan, 7-10June, 1993, pp.888-891.[25] F. Cat'dot, J. Gohet, M. Boganski and F. Rudolf, Microfabrication of tdgh-density arrays for micro electromagnets wlth on chip electronics, Tech. Digest, 7th int. Conf. Solid-State Sensors and Actuators (Transducers "93), Yokohamo. Japan, 7-10June, 1993, pp. 32-35.[26] W. Tang, V. Temesvary, JJ. YBO, Y.C. Tat and D.K. Miu, Siliconmicroactuators for computer disk drives, Jpn. J. Appl. Phys., 35 (1996).~50-356.[27] D. Miu and Y.C. Tai, Scaled technology: 10 GB/in 2. Workshop on MEMS. Taipai. Tmwan. 21-24 March. 1994. p. 36.译文:微机电系统的未来(MEMS)Minhang Bao a, Weiyuan Wang b• Fudan University, Shanghai. 200433 Chinab Shanghai Institute of Metallurgy. Shanghai. 200050 China摘要基于微加工和微电子技术微机电系统(MEMS)的发展已经近十年显著。
- 1、下载文档前请自行甄别文档内容的完整性,平台不提供额外的编辑、内容补充、找答案等附加服务。
- 2、"仅部分预览"的文档,不可在线预览部分如存在完整性等问题,可反馈申请退款(可完整预览的文档不适用该条件!)。
- 3、如文档侵犯您的权益,请联系客服反馈,我们会尽快为您处理(人工客服工作时间:9:00-18:30)。
The most important figures of merit of the microcapacitor
第一届微纳米企业研讨会(无锡) 第一届微纳米企业研讨会(无锡) 方东明 May 10, 2008
微电子学研究院
微米纳米加工技术国家级重点实验室
Introduction
Table II Classification of the tunable capacitor Shape of plate Drive ways Move ways parallel plates two plates three plates heat electrostatic piezoelectric vertical
Meander
Type Performance
Spiral planar vertical Suspended 4 35
Solenoid Aircore 3 >30 Magn eticcore 30 10
planar
vertical
Inductance (nH) Quality Factor Resonant Frequency (GHz)
Insulated material
Sacrificial material
Geometrical parameters
第一届微纳米企业研讨会(无锡) 第一届微纳米企业研讨会(无锡)
方东明
May 10, 2008
微电子学研究院
微米纳米加工技术国家级重点实验室
Introduction
Three types of MEMS inductor
第一届微纳米企业研讨会(无锡) 第一届微纳米企业研讨会(无锡)
s:space between conductors N:turn : :
第一届微纳米企业研讨会(无锡) 第一届微纳米企业研讨会(无锡) 方东明 May 10, 2008
微电子学研究院
微米纳米加工技术国家级重点实验室
Fabrication
substrate seed layer mask photoresist copper
Outline
Introduction Simulation
Outline
Fabrication Results Conclusion
第一届微纳米企业研讨会(无锡) 第一届微纳米企业研讨会(无锡)
方东明
May 10, 2008
微电子学研究院
微米纳米加工技术国家级重点实验室
Background
Wireless communication Mobile communication Global Position System
The most important figures of merit of the microinductor
第一届微纳米企业研讨会(无锡) 第一届微纳米企业研讨会(无锡) 方东明 May 10, 2008
微电子学研究院
微米纳米加工技术国家级重点实验室
Introduction
TableⅠ Comparison of the three types of MEMS inductors Ⅰ
Results
Why our fabricated solenoid inductors have high Q-factors?
eddy current loss is potentially minimal Solenoid-type inductor decrease the skin effect and ohmic loss Low resistivity metal (copper) decrease the dielectric loss SiO2 decrease the substrate loss High resistivity substrate 第一届微纳米企业研讨会(无锡) 第一届微纳米企业研讨会(无锡) 方东明 May 10, 2008
微电子学研究院
微米纳米加工技术国家级重点实验室
Introduction
Applications of microinductors and microcapapcitors Non-contact IC card Radio-broadcast transmitter
Filter
Microwave field
comb piezioresist electromagnetic angle
horizontal
第一届微纳米企业研讨会(无锡) 第一届微纳米企业研讨会(无锡)
方东明
点实验室
Simulation
……
Save time
Reduce cost
Ansys: : simulate the movable structure
第一届微纳米企业研讨会(无锡) 第一届微纳米企业研讨会(无锡)
方东明
May 10, 2008
微电子学研究院
微米纳米加工技术国家级重点实验室
Simulation
Results
HFSS Simulation
Build model Set material
small small
small improved
small 5
1 24
1
5.5
6
>3
0.5
第一届微纳米企业研讨会(无锡) 第一届微纳米企业研讨会(无锡)
方东明
May 10, 2008
微电子学研究院
微米纳米加工技术国家级重点实验室
Introduction
Three types of MEMS capacitor
微电子学研究院
微米纳米加工技术国家级重点实验室
Conclusion
The fabrication process …
The measured results …
Integrating …
第一届微纳米企业研讨会(无锡) 第一届微纳米企业研讨会(无锡)
方东明
May 10, 2008
fangdm@
l(m) w(m) t(m) 350 350 250 350 50 100 100 50 5 5 5 5
s(m) h(m) 40 40 40 40 45 15 15 15
第一届微纳米企业研讨会(无锡) 第一届微纳米企业研讨会(无锡)
方东明
May 10, 2008
微电子学研究院
微米纳米加工技术国家级重点实验室
Systems On Chip
Low cost
第一届微纳米企业研讨会(无锡) 第一届微纳米企业研讨会(无锡)
方东明
May 10, 2008
微电子学研究院
微米纳米加工技术国家级重点实验室
Introduction
Metal
Process
Substrate
Main factors affecting the performance of inductors and capacitors
Simulation
Estimate performance
第一届微纳米企业研讨会(无锡) 第一届微纳米企业研讨会(无锡)
方东明
May 10, 2008
微电子学研究院
微米纳米加工技术国家级重点实验室
Simulation
Simulation
HFSS: simulate the performance at high frequency
Port/ Boundary condition Grid/ Frequency condition
第一届微纳米企业研讨会(无锡) 第一届微纳米企业研讨会(无锡)
方东明
May 10, 2008
微电子学研究院
微米纳米加工技术国家级重点实验室
Simulation
Fig.1. HFSS model of the inductor and the capacitor
Resonator
Oscillator
第一届微纳米企业研讨会(无锡) 第一届微纳米企业研讨会(无锡)
Power amplifier
方东明 May 10, 2008
微电子学研究院
微米纳米加工技术国家级重点实验室
Introduction
Low weight Small size Low power
Multifunction Highly integrated
Fig.4. The fabrication process …
第一届微纳米企业研讨会(无锡) 第一届微纳米企业研讨会(无锡)
方东明
May 10, 2008
微电子学研究院
微米纳米加工技术国家级重点实验室
Result
Table III The geometrical …
N Group A Group B Group C Group D 7 7 7 7
High frequency High performance
Capacitor Inductor
Performance IC Size Cost
Q-factor Inductance Resonance frequency
Our desired goal
第一届微纳米企业研讨会(无锡) 第一届微纳米企业研讨会(无锡) 方东明 May 10, 2008
Surface micromachined RF MEMS solenoid inductors and variable capacitors Dong-Ming Fang
May 10, 2008 第一届微纳米企业研讨会(无锡) 第一届微纳米企业研讨会(无锡)
微电子学研究院
微米纳米加工技术国家级重点实验室