Networks on Chips A Synthesis Perspective
ccna第二章答案
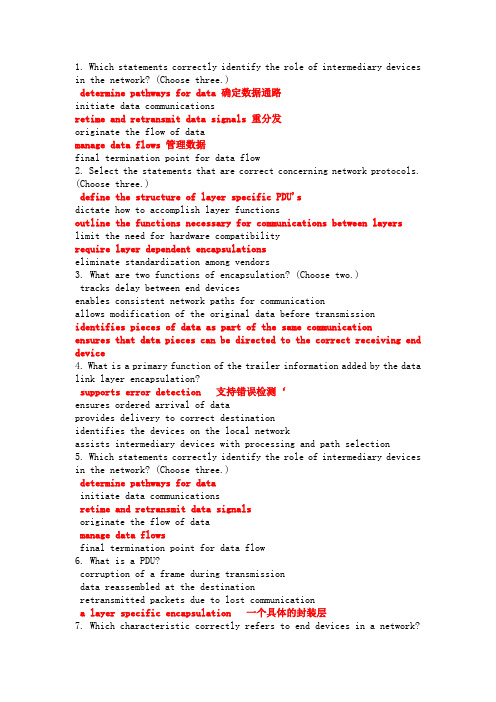
1. Which statements correctly identify the role of intermediary devices in the network? (Choose three.)determine pathways for data 确定数据通路initiate data communicationsretime and retransmit data signals 重分发originate the flow of datamanage data flows 管理数据final termination point for data flow2. Select the statements that are correct concerning network protocols. (Choose three.)define the structure of layer specific PDU'sdictate how to accomplish layer functionsoutline the functions necessary for communications between layers limit the need for hardware compatibilityrequire layer dependent encapsulationseliminate standardization among vendors3. What are two functions of encapsulation? (Choose two.)tracks delay between end devicesenables consistent network paths for communicationallows modification of the original data before transmissionidentifies pieces of data as part of the same communicationensures that data pieces can be directed to the correct receiving end device4. What is a primary function of the trailer information added by the data link layer encapsulation?supports error detection 支持错误检测‘ensures ordered arrival of dataprovides delivery to correct destinationidentifies the devices on the local networkassists intermediary devices with processing and path selection5. Which statements correctly identify the role of intermediary devices in the network? (Choose three.)determine pathways for datainitiate data communicationsretime and retransmit data signalsoriginate the flow of datamanage data flowsfinal termination point for data flow6. What is a PDU?corruption of a frame during transmissiondata reassembled at the destinationretransmitted packets due to lost communicationa layer specific encapsulation 一个具体的封装层7. Which characteristic correctly refers to end devices in a network?manage data flowsoriginate data flow 原数据流retime and retransmit data signalsdetermine pathways for data8.Refer to the exhibit. "Cell A" at IP address 10.0.0.34 has established an IP session with "IP Phone 1" at IP address 172.16.1.103. Based upon the graphic, which device type best describes the function of wireless device "Cell A?"the destination devicean end devicean intermediate devicea media device9.Refer to the exhibit. Which three labels correctly identify the network types for the network segments that are shown?(Choose three.)Network A -- WANNetwork B -- WANNetwork C -- LANNetwork B -- MANNetwork C -- WANNetwork A -- LAN10. Which three statements best describe a Local Area Network (LAN)? (Choose three.)A LAN is usually in a single geographical area.The network is administered by a single organization.The connection between segments in the LAN is usually through a leased connection.The security and access control of the network are controlled by a service provider.A LAN provides network services and access to applications for users within a common organization.Each end of the network is generally connected to a Telecommunication Service Provider (TSP).11.Refer to the exhibit. Which networking term describes the data interleaving process represented in the graphic?pipingPDUstreamingmultiplexingencapsulation12. What is the primary purpose of Layer 4 port assignment?to identify devices on the local mediato identify the hops between source and destinationto identify to the intermediary devices the best path through the network to identify the source and destination end devices that are communicating to identify the processes or services that are communicating within the end devices13. What device is considered an intermediary device?1.file serve2.IP phone ptop 4.printer 5.switch14.Refer to the exhibit. Which term correctly identifies the device type that is included in the area B? sourceendtransferintermediary 中间设备15.Refer to the exhibit. What type of network is shown?WANMANLANWLAN16. Which layer encapsulates the segment into packets?physicaldata linknetwork 网络层封装的是数据包transport17. What can be identified by examining the network layer header?the destination device on the local mediathe destination host address 网络层头部封装目的主机的地址the bits that will be transferred over the mediathe source application or process creating the data18. What is the purpose of the TCP/IP Network Access layer?path determination and packet switchingdata presentationreliability, flow control, and error detectionnetwork media control 接入层控制网络媒体the division of segments into packets19. During the encapsulation process, what occurs at the data link layer? No address is added.The logical address is added.The physical address is added. 数据链路层添加一个物理地址The process port number is added.20.Refer to the exhibit. Which set of devices contains only end devices? A, C, DB, E, G, HC, D, G, H, I, JD, E, F, H, I, JE, F, H, I, J21. What is the proper order of the layers of the OSI model from the highest layer to the lowest layer?4physical, network, application, data link, presentation, session, transportapplication, physical, session, transport, network, data link, presentationapplication, presentation, physical, session, data link, transport, networkapplication, presentation, session, transport, network, data link, physicalpresentation, data link, session, transport, network, physical, application22. Which two layers of the OSI model have the same functions as the TCP/IP model Network Access Layer? (Choose two.)34NetworkTransportPhysicalData Link TCP/IP 工作在第一层和第二层Session。
半导体方向英文普刊
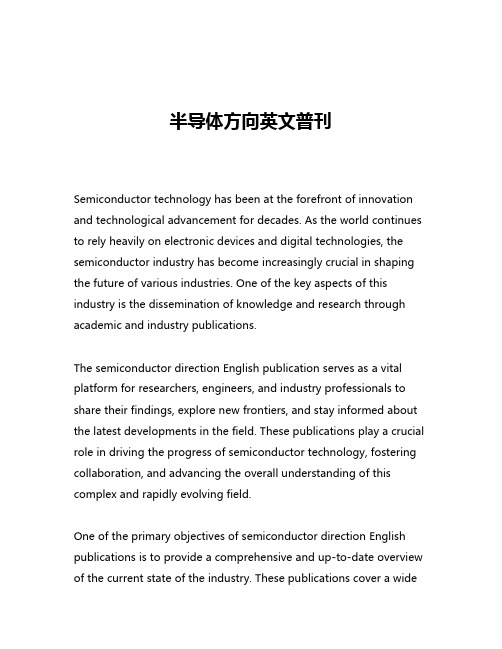
半导体方向英文普刊Semiconductor technology has been at the forefront of innovation and technological advancement for decades. As the world continues to rely heavily on electronic devices and digital technologies, the semiconductor industry has become increasingly crucial in shaping the future of various industries. One of the key aspects of this industry is the dissemination of knowledge and research through academic and industry publications.The semiconductor direction English publication serves as a vital platform for researchers, engineers, and industry professionals to share their findings, explore new frontiers, and stay informed about the latest developments in the field. These publications play a crucial role in driving the progress of semiconductor technology, fostering collaboration, and advancing the overall understanding of this complex and rapidly evolving field.One of the primary objectives of semiconductor direction English publications is to provide a comprehensive and up-to-date overview of the current state of the industry. These publications cover a widerange of topics, including materials science, device design, fabrication processes, circuit design, and system-level integration. By showcasing the latest research and innovations, these publications help to shape the direction of the semiconductor industry and inspire further advancements.Moreover, semiconductor direction English publications serve as a platform for the exchange of ideas and the dissemination of knowledge. Researchers and engineers from around the world contribute their research findings, case studies, and innovative solutions to these publications, allowing for the cross-pollination of ideas and the identification of emerging trends. This collaborative approach is essential in driving the semiconductor industry forward, as it enables the sharing of best practices, the identification of challenges, and the exploration of new avenues for exploration and development.One of the key strengths of semiconductor direction English publications is their ability to provide a global perspective on the industry. These publications often feature articles and research from diverse geographical regions, allowing readers to gain insights into the different approaches, challenges, and successes experienced in various parts of the world. This global outlook is particularly valuable in an industry that is inherently international, with supply chains, manufacturing facilities, and research centers spread across theglobe.In addition to disseminating research and technical knowledge, semiconductor direction English publications also play a crucial role in shaping the future of the industry. These publications often feature thought-leadership articles, industry analyses, and strategic insights that help to inform decision-makers, policymakers, and industry stakeholders. By providing a platform for the discussion of emerging trends, disruptive technologies, and long-term industry prospects, these publications contribute to the strategic planning and decision-making processes within the semiconductor industry.One of the key challenges facing the semiconductor direction English publication landscape is the rapid pace of technological change. The semiconductor industry is characterized by constant innovation, with new materials, device architectures, and manufacturing processes emerging at a breakneck pace. Keeping up with these advancements and ensuring the timely dissemination of relevant and accurate information is a significant challenge for these publications.To address this challenge, semiconductor direction English publications have had to adapt and evolve their editorial and publishing strategies. This has often involved the adoption of digital platforms, the use of data analytics to identify emerging trends, and the development of specialized content streams to cater to thediverse needs of their readership. Additionally, these publications have had to maintain a strong focus on quality, accuracy, and relevance, ensuring that the information they provide is both cutting-edge and reliable.Another challenge facing semiconductor direction English publications is the need to balance the technical depth and rigor required by the industry with the need to communicate complex concepts in an accessible and engaging manner. Semiconductor technology is inherently complex, with a high degree of technical jargon and specialized knowledge. Translating this technical information into a format that is understandable and relevant to a broad audience of industry professionals, researchers, and policymakers is a significant challenge that these publications must navigate.To address this challenge, semiconductor direction English publications have had to develop innovative editorial strategies, such as the use of visual aids, case studies, and industry-specific language guides. They have also had to cultivate a pool of highly skilled writers and editors who are capable of translating complex technical concepts into clear and compelling narratives.Despite these challenges, semiconductor direction English publications continue to play a vital role in the advancement of thesemiconductor industry. By providing a platform for the dissemination of knowledge, the exchange of ideas, and the exploration of emerging trends, these publications have become essential resources for industry professionals, researchers, and policymakers alike.Looking to the future, it is clear that the importance of semiconductor direction English publications will only continue to grow. As the semiconductor industry continues to evolve and shape the technological landscape, the need for high-quality, authoritative, and forward-looking publications will become increasingly critical. These publications will be called upon to not only report on the latest developments, but also to provide strategic insights, thought leadership, and a global perspective on the industry's trajectory.In conclusion, semiconductor direction English publications are essential components of the semiconductor industry, serving as vital platforms for the dissemination of knowledge, the exchange of ideas, and the exploration of emerging trends. Despite the challenges posed by the rapid pace of technological change and the need to balance technical depth with accessibility, these publications continue to play a crucial role in driving the progress of the semiconductor industry and shaping its future direction. As the world becomes increasingly reliant on electronic devices and digital technologies, the importance of these publications will only continueto grow, making them indispensable resources for industry professionals, researchers, and policymakers alike.。
英语作文-集成电路设计行业的智能芯片与系统解决方案
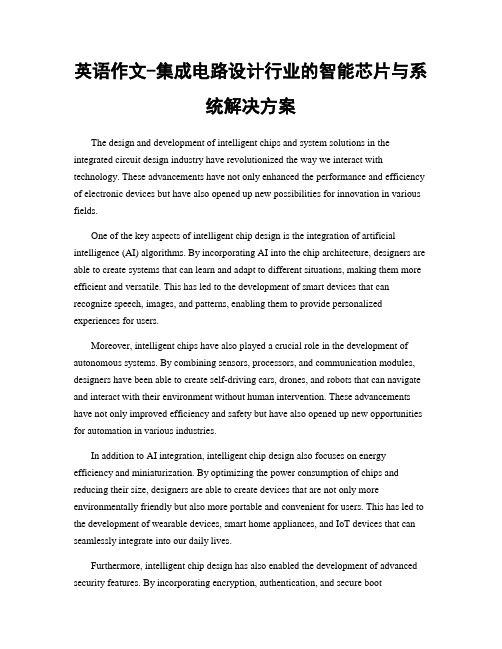
英语作文-集成电路设计行业的智能芯片与系统解决方案The design and development of intelligent chips and system solutions in the integrated circuit design industry have revolutionized the way we interact with technology. These advancements have not only enhanced the performance and efficiency of electronic devices but have also opened up new possibilities for innovation in various fields.One of the key aspects of intelligent chip design is the integration of artificial intelligence (AI) algorithms. By incorporating AI into the chip architecture, designers are able to create systems that can learn and adapt to different situations, making them more efficient and versatile. This has led to the development of smart devices that can recognize speech, images, and patterns, enabling them to provide personalized experiences for users.Moreover, intelligent chips have also played a crucial role in the development of autonomous systems. By combining sensors, processors, and communication modules, designers have been able to create self-driving cars, drones, and robots that can navigate and interact with their environment without human intervention. These advancements have not only improved efficiency and safety but have also opened up new opportunities for automation in various industries.In addition to AI integration, intelligent chip design also focuses on energy efficiency and miniaturization. By optimizing the power consumption of chips and reducing their size, designers are able to create devices that are not only more environmentally friendly but also more portable and convenient for users. This has led to the development of wearable devices, smart home appliances, and IoT devices that can seamlessly integrate into our daily lives.Furthermore, intelligent chip design has also enabled the development of advanced security features. By incorporating encryption, authentication, and secure bootmechanisms into the chip architecture, designers are able to create systems that can protect sensitive data and prevent unauthorized access. This has become increasingly important in today's interconnected world, where cyber threats are becoming more sophisticated and prevalent.Overall, the integration of intelligent chips and system solutions in the integrated circuit design industry has transformed the way we interact with technology. From AI-powered devices to autonomous systems and energy-efficient gadgets, these advancements have not only improved the performance and efficiency of electronic devices but have also opened up new possibilities for innovation in various fields. As technology continues to evolve, intelligent chip design will play a crucial role in shaping the future of electronics and revolutionizing the way we live and work.。
神经网络专题ppt课件
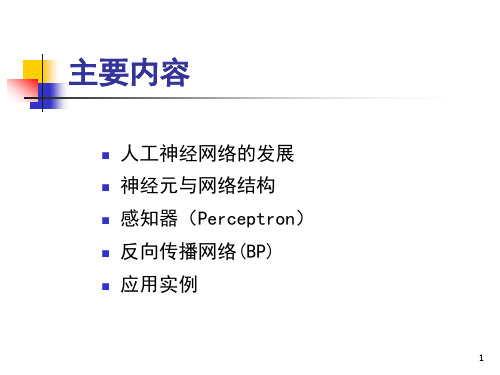
(4)Connections Science
(5)Neurocomputing
(6)Neural Computation
(7)International Journal of Neural Systems
7
3.2 神经元与网络结构
人脑大约由1012个神经元组成,而其中的每个神经元又与约102~ 104个其他神经元相连接,如此构成一个庞大而复杂的神经元网络。 神经元是大脑处理信息的基本单元,它的结构如图所示。它是以细胞 体为主体,由许多向周围延伸的不规则树枝状纤维构成的神经细胞, 其形状很像一棵枯树的枝干。它主要由细胞体、树突、轴突和突触 (Synapse,又称神经键)组成。
15
4.互连网络
互连网络有局部互连和全互连 两种。 全互连网络中的每个神经元都 与其他神经元相连。 局部互连是指互连只是局部的, 有些神经元之间没有连接关系。 Hopfield 网 络 和 Boltzmann 机 属于互连网络的类型。
16
人工神经网络的学习
学习方法就是网络连接权的调整方法。 人工神经网络连接权的确定通常有两种方法:
4
5. 20世纪70年代 代表人物有Amari, Anderson, Fukushima, Grossberg, Kohonen
经过一段时间的沉寂后,研究继续进行
▪ 1972年,芬兰的T.Kohonen提出了一个与感知机等神经 网络不同的自组织映射理论(SOM)。 ▪ 1975年,福岛提出了一个自组织识别神经网络模型。 ▪ 1976年C.V.Malsburg et al发表了“地形图”的自形成
6
关于神经网络的国际交流
第一届神经网络国际会议于1987年6月21至24日在美国加州圣地亚哥 召开,标志着神经网络研究在世界范围内已形成了新的热点。
超级智能——大脑芯片(英文)
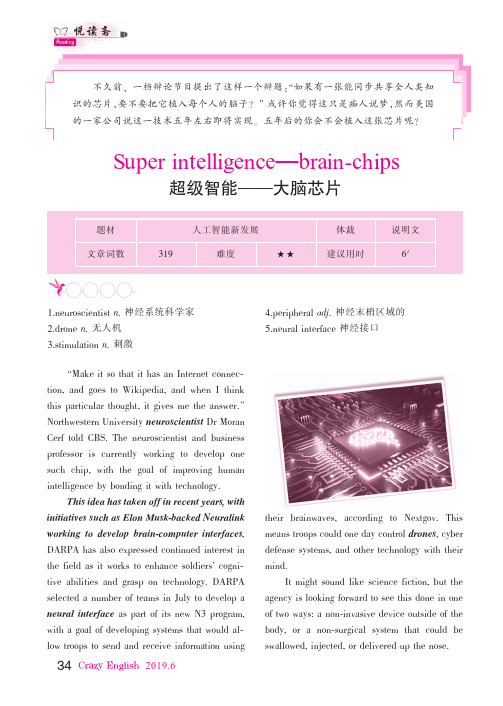
34 Crazy English 2019.6
their brainwaves, according to Nextgov. This means troops could one day control drones, cyber defense systems, and other technology with their mind.
不久前, 一档辩论节目提出了这样一个辩题:“如果有一张能同步共享全人类知 识的芯片,要不要把它植入每个人的脑子? ” 或许你觉得这只是痴人说梦,然而美国 的一家公司说这一技术五年左右即将实现。 五年后的你会不会植入这张芯片呢?
Super intelligence—brain-chips
一种高吞吐低延迟片上互连网络路由器

第50 卷第 8 期2023年8 月Vol.50,No.8Aug. 2023湖南大学学报(自然科学版)Journal of Hunan University(Natural Sciences)一种高吞吐低延迟片上互连网络路由器李晋文†,申慧毅,齐树波(国防科技大学计算机学院,湖南长沙 410073)摘要:本文提出了一种用于片上互连网络的低延迟高吞吐量动态虚拟输出队列路由器,该路由器可以利用前瞻路由计算和虚拟输出队列方案将路由器延迟减低到两个周期.仿真结果表明,与虫孔路由器和虚通道路由器相比,4×4网格上的网络吞吐量分别提高了46.9%和28.6%,并且在相同输入加速比下,性能比双缓冲虚通道路由器要高1.9%.在随机合成流量下,片上网络的零负载延迟也分别降低了25.6%和41%.设计实现结果表明,路由器的工作频率可以达到2.5 GHz.关键词:片上网络;路由器;吞吐量;延迟中图分类号:TN913.3 文献标志码:AA High-throughpur Low-latency Router for On-chip InterconnectNetworksLI Jinwen†,SHEN Huiyi,QI Shubo(School of Computer Science, National University of Defense Technology, Changsha 410073, China)Abstract:A low-latency high-throughput Dynamic Virtual Output Queues Router for On-chip interconnect networks is proposed in this paper,which can reduce the router latency to two cycles by leveraging look-ahead routing computation and virtual output queues scheme. The simulation results show that,compared with the wormhole router and virtual-channel router, the network throughput on a 4×4 mesh increases by up to 46.9% and 28.6%, respectively, and outperforms doubled buffer virtual channel by 1.9% under the same input speedup. Under random synthetic traffic,the zero-load-latency of the network on chip is also reduced by 25.6% and 41%,respectively. Synthesis results indicate the frequency of router can reach 2.5 GHz.Key words:on-chip network;router;throughput;latency随着半导体技术的飞速发展,越来越多的处理器核(多核和众核)集成在单个芯片上,而随着MOS 管尺寸的不断缩小,门级电路延迟在不断缩小,全局互连线的延迟相对于MOS管延迟还在不断增加.微∗收稿日期:2022-11-03基金项目:HPCL国家重点实验室基金项目(202101-02);国家自然科学基金资助项目(60873212),National Natural Science Foundation of China(60873212)作者简介:李晋文(1975—),男,山西武乡人,国防科技大学研究员,博士† 通信联系人,E-mail:*****************文章编号:1674-2974(2023)08-0141-06DOI:10.16339/ki.hdxbzkb.2023289湖南大学学报(自然科学版)2023 年处理器体系结构设计的重点正在从以提高计算为中心的单核能力设计转向以互连通信为中心的多核设计.由于互连延迟可预测、设计复杂度比较低、易扩展性和结构规整,片上网络已成为CMP和MPSoC中片上众核互连最有前途的选择[1].其中2D mesh互连网络已广泛应用于许多原型芯片,如Intel 80核Tera⁃flop、Tilera 64核和TRIPS[2-4].片上网络的概念来源于多处理器间互连网络,但实际与多芯片间互连网络有着许多不同的特点.最重要的一点,芯片内互连线和引脚比芯片间网络中的互连线和引脚资源更丰富[1].然而,片上网络中缓冲buffer容量不足.网络的延迟对实际多核的计算性能有很大影响.当路由器的每跳延迟从一个周期增加到五个周期时,全系统的性能将下降10%[5].基准的虚通道路由器的流水线级数为4.近年来,业界提出了几种新型架构的低延迟路由器,包括推测虚通道路由器[6]、采用虫孔交换的两虚通道结构路由器[7]、混合电路交换路由器[5]、带bundle的两周期路由器[8]、组合型两周期路由器[9]、无缓存片上路由器[10]、基于时间序列开关分配路由器[11]以及关键路径延迟只有35个FO4[12]的单周期路由器(FO4是指一个反相器驱动四个相同尺寸反相器产生的延迟,高性能微处理器的周期一般约为20个FO4).缓冲buffer的实现对互连网络的性能至关重要.缓冲buffer可以用寄存器或SRAM来实现.在芯片中,通常缓冲buffer的容量相对较小,因此使用低延迟的寄存器实现更为有利,而使用SRAM会存在较大的地址译码延迟以及存储阵列访问延迟,这些延迟与全局位线相关;此外还能节省位线预充电功耗[13].在标准的虚通道路由器中,每个虚通道都需要自带缓冲buffer,一个虚通道无法使用其他虚通道的缓冲buffer[14].DAMQ路由器设立了5个缓冲buffer队列,每个队列对应一个虚通道,多出的一个队列作为共享缓冲buffer,一个报文flit从到达到离开路由器需要3个时钟周期[15].VichaR路由器能够根据数据流量(traffic)来调节和分配每个物理通道的虚通道和缓冲buffer数量,并使用复杂的VC控制表来管理报文flit,能够有效提高缓冲buffer的使用效率,其缺点是路由器延迟会达到四个时钟周期.当路由器中发生拥塞时,无论是采用基于信用还是基于开关的流控策略,通道流水线中的缓冲buffer都不能用于缓冲flit.iDEAL路由器提出用中继器(repeater)电路来缓冲flit报文[16],然而中继器存在较大漏流问题,会导致不可靠.本文提出了一种新型的两周期路由器——动态虚通道输出队列路由器(DVOQR),采用多端口缓冲buffer和虚拟输出队列来消除虚通道路由器中的分配站(allocation stage).采用Ready/Valid握手机制来控制路由器之间的flit流,在这种策略下,流水线通道中的存储器可以用于缓冲flit报文.本文其余部分组织如下,第1节介绍了路由器的微架构.第2节给出了路由器的具体设计实现.第3节分析了模拟结果.最后,第4节对本文工作进行了简要总结.1 路由器微架构1.1 DVOQR路由器微架构本文提出了一种新型动态虚通道输出队列路由器(DVOQR),其微架构如图1所示.路由器包括P个输入端口和P个输出端口.对于二维mesh网络,P= 5;一个端口连接到本地处理器(核),其他端口连接到相邻路由器.输入单元由三个主要模块组成:集中动态缓冲器(Unified Dynamic Buffer,UDB)、集中动态缓冲分配器(Unified Dynamic Buffer Allocation,UDBA)、P个虚拟输出地址队列(Virtual Ouput Address Queue,以下简称VOAQ).输出端口包括一个P选1的仲裁器和一个P输入的多路复用器.由多个flit组成1个数据报文,存储在同一FIFO队列中,路由到同一输出端口.每个输入端口有P个FIFO队列,它们共享一个UDB并各自带一个私有的VOAQ.每个FIFO中flit的地址存储在虚拟输出地址队列(VOAQ)中.这样一来,就可以有效消除队列头阻塞(HOL)延迟问题[17].芯片间网络路由器中的缓冲buffer一般使用SRAM来实现.大容量的多端口SRAM存储器由于需要较大的面积开销、较高的功耗和访问延迟而难以实现,而使用小容量的寄存器来实现多端口缓冲器buffer要容易得多.受片上资源的限制,UDB用低延迟的多端口寄存器实现,具有1个写端口和P个读端口.每个读端口对应1个FIFO队列.尽管使用多个端口会导致面积开销增加,但可以消除虚通道路由器流水线的分配站.连接到输出端口的CDB,由CDB控制器和两项142第 8 期李晋文等:一种高吞吐低延迟片上互连网络路由器寄存器组成,如图2(a )所示.其中一个寄存器负责接收来自路由器的flit ,而另一个寄存器负责将flit 发送到下一个路由器,一收一发.在下一个周期中,两个寄存器交换收发功能.因此CDB 可以同时接收和发送flit ,可以避免流水线产生气泡.图2(b )给出了CDB 控制器的实现电路.state [1:0]表征两个寄存器的状态.读指针rd_ptr 对应发送寄存器,写指针wr_ptr 对应接收寄存器.当路由器之间的线延迟超过一个时钟周期时,可以插入多个CDB.UDBA 用于为队列分配时隙或释放空时隙.使用状态向量来跟踪所有时隙的状态,1表示时隙可用.当时隙分配给flit 时,相应的位将被清掉.采用固定优先级仲裁器以简化分配逻辑,最低可用时隙将被分配最高的优先级.设计了四个物理VOAQ 来缓存同一队列中的flit.当某一个flit 注入UDB 时,UDBA 负责将分配给它的时隙号写入对应的VOAQ ,该VOAQ 还会保存该报文的路由信息以及flit 类型.在UDB 读操作之前,需要首先从VOAQ 中读出UDB 中flit 的地址,这将增加UDB 的访问延迟.本文设计了一种新颖的移位FIFO ,可以有效减少UDB 的读延迟.图3给出了VOAQ 的微架构,使用one-hot 向量来指向FIFO 的尾部,而第一项指向FIFO 的头部.尾向量的宽度比UDB 的深度D 要大1.当tail_vector [0]为1时,FIFO 为空;而tail_vector [D ]等于1时,FIFO 为满.当头数图1 DVOQR 路由器微架构Fig.1 Microarchitecture of DVOQR(a )Architecture of channel double buffer(b ) Channel double buffer controller图2 通道的双缓存控制器Fig.2 Channel double buffer controller143湖南大学学报(自然科学版)2023 年据离开队列时,VOAQ 中的其他数据将向前移一位,而tail_vector 将进行右移.当新数据到达时,数据将被添加到VOAQ 的尾部,并且tail_vector 左移1位.当新数据在同一时钟周期内到达和离开时,tail_vector 将不发生移位.DVOQR 中的交换分配单元使用P 个round-robin 仲裁器实现.交换分配单元只需要一级仲裁,即可实现最大匹配,从而提高路由器吞吐量并降低分配延迟.1.2 DVOQR 流水线设计DVOQR 路由器的流水线由两站组成:flit 交换站(Flit Switch ,FS )和链路传输站(Link Traversal ,LT ).FS 站:完成交叉开关分配、前瞻路由计算、UDB读操作和Crossbar 传输.其中交叉开关分配、前瞻路由计算和UDB 读操作能够并行.当VOAQ 的第一项是head flit 报文片时,会为目的仲裁器产生一个请求信号.同时,发送VOAQ 中的flit 地址到UDB ,启动读操作,根据报文的路由信息,采用维序路由算法进行路由的前瞻计算.如果请求未被批准,将在下一个周期中重试,而不需要再次读取flit 报文.LT 站:在这一站中,flit 通过物理链路发送并写入UDB ,并根据FS 站的前瞻路由计算结果,将分配给flit 的地址写入VOAQ 中.1.3 流控机制DVOQR 使用了一种新的流控机制,称为ready-valid 握手机制(handshake ).ready 输出表示UDB/CDB 有可用的存储来接收flit 报文.valid 信号标识当前的flit 报文是有效的.当ready 和valid 信号在同一个周期内有效时,说明flit 报文已经提交.当下一级路由器发生拥塞时,链路上流水线中的CDB 可以缓冲flit 报文,这等效于增加了缓冲buffer 容量.基于维序路由算法,这种流控机制可以有效避免死锁.2 设计实现基于RTL 设计实现了用于片上2D mesh 网络的DVOQR 路由器,数据位宽128位,带有16项UDB ,评估了路由器的性能和功耗,综合生成门级网表,并对时序进行了详细的分析.FS 站和LT 站的关键路径延迟分别为400 ps (11.4 FO4)和252 ps (7.2 FO4),该工艺下的FO4为35 ps.表1给出了路由器中各功能部件的面积和功耗.3 模拟结果3.1 模拟方法本文采用随机人工合成流量模型评估互连网络的性能.表2给出了模拟实验的参数设置.采用周期精确模拟器Booksim [14]来评估虫孔路由器(Worm⁃hole Router ,WH )和虚通道路由器(Virtual-channel Router ,VC ).本文使用Verilog HDL 设计实现了DVOQR 的RTL 模型.测试程序采用随机通讯的合成程序,进行了仿真模拟,预热时间为1万个时钟周期,测量时间为10万个时钟周期.3.2 模拟结果分析3.2.1 不同缓冲容量的影响图4为带16项UDB 的DVOQR 路由器在随机流量负载下的平均延迟曲线.虫孔路由器和虚信道路由器中的输入缓冲buffer 数量为16~64 flit.与其他两种路由器相比,DVOQR 的吞吐量分别增加了33.2%和12%,而其他路由器缓冲buffer 的容量是DVOQR 的3倍.因此,DVOQR 可以更有效地使用输入缓冲器.其中,三种路由器的零负载延迟分别为10.4、14.0和17.7.表1 路由器中各功能部件的面积和功耗Tab.1 Area and power consumption of each functionalcomponent模块UDBVOAQinput portoutput port CDBrouter 组合逻辑面积/(μm )218 9452 49629 7311 5102 236167,385时序逻辑面积/(μm )231 47531 6844 0931133 065221,595总面积/(μm )250 4205 66473 8241 6235 301403,740功耗/mW58.87.589.30.60312.1507.5数量/个5205551图3 VOAQ 的微架构Fig.3 Microarchitecture of virtual ouput address queue144第 8 期李晋文等:一种高吞吐低延迟片上互连网络路由器3.2.2 相同输入加速比UDB 有四个读端口,因此DVOQR 的输入加速比是4.图5给出了在随机流量负载相同输入加速比时的平均延迟曲线.与VC_4×4和VC_4×8相比,VOQ_16的吞吐率分别增加17.6% 和1.9%,而VC_8×8 和VC_8×16的吞吐率分别比VOQ_16要高2.9%和7.5%.DVOQR 吞吐率比双缓冲虚通道路由器要高1.9%.在相同的输入加速比下,采用动态缓冲buffer分配只需要一半的buffer 容量就能达到相同的吞吐率.3.2.3 UDB 深度的影响图6给出了随机流量下DVOQR 网络性能与UDB 深度的相关性.2项UDB 的网络饱和点约为50%,16项UDB 的饱和点可达到82.4%.当UDB 的深度大于8时,吞吐率的增加随着UDB 深度的增加速度放缓.当注入流量小于0.4时,采用不同深度UDB 的平均延迟几乎是相同的.可以根据网络流量打开或关闭一部分UDB ,这样可以有效减少缓冲buffer 的漏流功耗.事实上,缓冲buffer 产生的漏流功耗是整个NoC 路由器漏流功耗的最主要来源.3.2.4 报文长度的影响图7给出了随机流量下带16项UDB 的DVOQR平均延迟与数据报文长度的关系,报文长度为2~32个flit.吞吐率随着报文长度的增加而降低.报文长度为32 flit 和2 flit 网络的饱和点分别为57.5%和87.5%.报文长度进一步增加将导致阻塞,因此需要占用更多的物理通道,而且竞争增加将导致更大的延迟.图7 对应不同报文长度下DVOQR 平均延迟Fig.7 Average latency of DVOQR under differentmessage lengths表2 模拟参数设置Tab.2 Simulation parameter settingsnetwork路由算法报文长度流量注入DVOQR 路由器虫孔路由器(WH )虚通道路由器(VC )4×4 meshdimension-order routing four flitsBernoulli processtwo-stage pipeline ,the depth of UDB is 16 for VOQ_16three-stage pipeline ,the depth of buffer is 16 for WH_16.four-stage pipeline ,the channel number is 4 and the depth of buffer in channel is 8for VC_4×8.图4 不同buffer 容量的DVOQR 路由器平均延迟Fig.4 Average latency of DVOQR with different buffer capacities图5 相同输入加速比下DVOQR 平均延迟Fig.5 Average latency of DVOQR under the sameinput acceleration ratio图6 不同深度UDB 的DVOQR 的平均延迟Fig.6 Average latency of DVOQR with different UDB145湖南大学学报(自然科学版)2023 年4 结论本文提出了一种基于ready-valid握手流控策略的两级流水线片上互连网络路由器,该路由器采用维序路由可以避免死锁.与虫孔路由器和虚通道路由器相比,4×4 mesh网络中的网络吞吐量分别提高了46.9%和28.6%,并且在相同的输入加速比下,DVOQR路由器比双缓冲虚通道路由器性能提高了1.9%.综合结果表明,路由器的时钟频率可达2.5 GHz.参考文献[1]DALLY W J,TOWLES B.Route packets,not wires:on-chip interconnection networks[C]//Proceedings of the 38th DesignAutomation Conference .Las Vegas,NV,USA:IEEE,2005:684-689.[2]VANGAL S,HOWARD J,RUHL G,et al.An 80-tile 1.28TFLOPS network-on-chip in 65nm CMOS[C]//2007 IEEE InternationalSolid-State Circuits Conference. San Francisco,CA,USA:IEEE,2007:98-589.[3]GRATZ P,KIM C,SANKARALINGAM K,et al.On-chip interconnection networks of the TRIPS chip[J].IEEE Micro,2007,27(5):41-50.[4]WENTZLAFF D,GRIFFIN P,HOFFMANN H,et al.On-chip interconnection architecture of the tile processor[J].IEEE Micro,2007,27(5):15-31.[5]JERGER N E,LIPASTI M,PEH L S.Circuit-switched coherence [J].IEEE Computer Architecture Letters,2007,6(1):5-8.[6]PEH L S,DALLY W J.A delay model and speculative architecture for pipelined routers[C]//Proceedings HPCA SeventhInternational Symposium on High-Performance ComputerArchitecture. Monterrey,Mexico:IEEE,2002:255-266.[7]胡哲琨,陈杰.消息传递型片上多核系统的设计[J].湖南大学学报(自然科学版),2013,40(8):102-109.HU Z K,CHEN J.Design of a message-passing multi-core system[J].Journal of Hunan University (Natural Sciences),2013,40(8):102-109.(in Chinese)[8]KUMARY A,KUNDUZ P,SINGHX A P,et al.A 4.6Tbits/s3.6GHz single-cycle NoC router with a novel switch allocator in65nm CMOS[C]//2007 25th International Conference onComputer Design. Lake Tahoe,CA,USA:IEEE,2008:63-70.[9]TIWARI V , KHARE K , SHANDILYA S . An efficient 4×4 mesh structure with a combination of two NoC router architecture[J].International Journal of Sensors,Wireless Communication andControl, 2021,11(2):169-180.[10]CHIOU S Y . Bufferless routing algorithms:a survey[J].Advances in Computational Sciences and Technology,2018,11(5):381-386.[11]李存禄,董德尊,吴际,等.低延迟路由器中高效开关分配机制的实现与评测[J].湖南大学学报(自然科学版),2015,42(4):78-84.LI C L,DONG D Z,WU J,et al.Design and implementation ofefficient switching in low-latency router[J].Journal of HunanUniversity (Natural Sciences),2015,42(4):78-84.(in Chinese)[12]MULLINS R,WEST A,MOORE S.The design and implementation of a low-latency on-chip network[C]//Proceedings of the 2006Asia and South Pacific Design Automation Conference.New York:ACM,2006:164-169.[13]HU J C,MARCULESCU R.Energy- and performance-aware mapping for regular NoC architectures[J].IEEE Transactions onComputer-Aided Design of Integrated Circuits and Systems,2005,24(4):551-562.[14]MULLINS R,WEST A,MOORE S.The design and implementation of a low-latency on-chip network[C]//Proceedings of the 2006Asia and South Pacific Design Automation Conference.New York:ACM,2006:164-169.[15]TAMIR Y,FRAZIER G L.High-performance multiqueue buffers for VLSI communication switches[C]//[1988]The 15th AnnualInternational Symposium on Computer Architecture.Honolulu,HI,USA: IEEE,2002:343-354.[16]KODI A,SARATHY A,LOURI A.Design of adaptive communication channel buffers for low-power area-efficientnetwork-on-chip architecture[C]//Proceedings of the 3rd ACM/IEEE Symposium on Architecture for Networking andCommunications Systems.New York:ACM,2007:47-56.[17]KAROL M,HLUCHYJ M,MORGAN S.Input versus output queueing on a space-division packet switch[J].IEEE Transactionson Communications,1987,35(12):1347-1356.146。
Network on chip
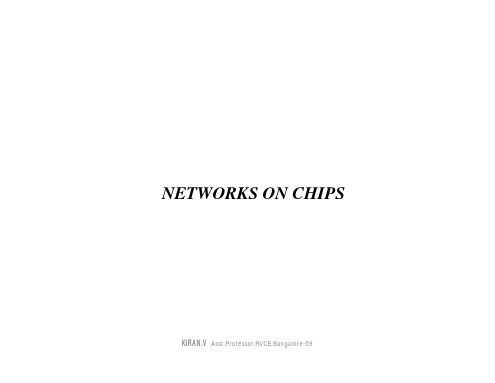
• PALM Chip ()
– M Bus/Palm Bus
• Mentor Graphics ()
– FISP Bus
• OMI (www.omimo.be)
– PI (peripheral Interconnect) Bus
KIRAN.V Asst.Professor.RVCE,Bangalore-59
Standard On-Chip-Buses
• ARM ()
– AMBA (Advanced Microcontroller Bus Architecture)
• IBM ()
批注本地保存成功开通会员云端永久保存去开通
NETWORKS ON CHIPS
KIRAN.V Asst.Professor.RVCE,Bangalore-59
Motivation
• Designing a system on a chip with large number of cores poses many challenging problems. • Designing a flexible on-chip communication network. • Designing a N/W which can provide the desired bandwidth and can be reused across many applications
KIRAN.V Asst.Professor.RVCE,Bangalore-59
• The communication architecture in such systems must also be able to support the Quality of service (QoS) • The needs of heterogeneous systems that will require multiple-modes of operations and with varying levels real time response requirements • The shrinking of process technology into the deepsubmicron (DSM) domain(i.e., below 90 nm)
周期信号傅里叶级数

分析公式 (正变换)
连续时间傅里叶级数对:
称为傅里叶系数或频谱系数
综合公式 (反变换)
3.三角形式傅立叶级数
若 f (t)为实函数,则有 利用这个性质可以将指数Fourier级数表示写为 令 由于C0是实的,所以b0=0,故 由此可以推出:
三角形式傅立叶级数
傅里叶系数 连续时间周期信号三角形式傅立叶级数为:
建议同学多看国外电子与通信教材系列 ,先看翻译版,再看英文硬印版
集成电路版图基础(英文影印版) (4小时出库)
Layout Basics:A Practical Guide
作者: CHRISTOPHER SAINT,JUDY SAINT
市场价: ¥45.00
模拟CMOS集成电路设计(英文影印版) (4小时出库) sign of Analog CMOS Integrated Circuits 作者: (美)BEHZAD RAZAVI 市场价: ¥68.00
四、周期信号的功率谱
周期信号属于功率信号,周期信号f(t)在1欧姆电阻上消耗的平均功率为:
单击此处添加小标题
由下面关系可以推导出,帕什瓦尔(Parseval)功率守恒定理:
单击此处添加小标题
01
02
四、周期信号的功率谱
物理意义:任意周期信号的平均功率等于信号所包含的直流、基波以及各次谐波的平均功率之和。
[解] 周期矩形脉冲的傅立叶系数为
将A=1,T=1/4,=1/20,w0=2p/T=8p 代入上式 功率谱
信号的平均功率为 包含在有效带宽(0~2p/t)内的各谐波平均功率为 周期矩形脉冲信号包含在有效带宽内的各谐波平均功率之和占整个信号平均功率的90%。
求f (t)的功率。
一种新的部分神经进化网络的股票预测(英文)
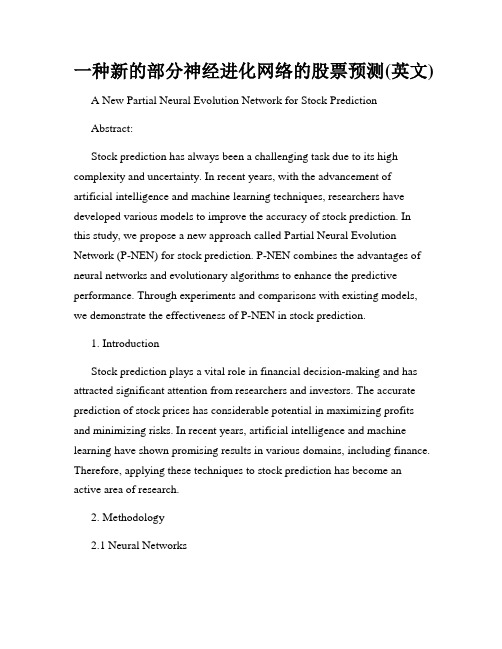
一种新的部分神经进化网络的股票预测(英文)A New Partial Neural Evolution Network for Stock PredictionAbstract:Stock prediction has always been a challenging task due to its high complexity and uncertainty. In recent years, with the advancement of artificial intelligence and machine learning techniques, researchers have developed various models to improve the accuracy of stock prediction. In this study, we propose a new approach called Partial Neural Evolution Network (P-NEN) for stock prediction. P-NEN combines the advantages of neural networks and evolutionary algorithms to enhance the predictive performance. Through experiments and comparisons with existing models, we demonstrate the effectiveness of P-NEN in stock prediction.1. IntroductionStock prediction plays a vital role in financial decision-making and has attracted significant attention from researchers and investors. The accurate prediction of stock prices has considerable potential in maximizing profits and minimizing risks. In recent years, artificial intelligence and machine learning have shown promising results in various domains, including finance. Therefore, applying these techniques to stock prediction has become an active area of research.2. Methodology2.1 Neural NetworksNeural networks have demonstrated great success in various fields, including pattern recognition and time series prediction. They are composed of interconnected artificial neurons and can learn complex patterns from historical data. In stock prediction, neural networks can capture the nonlinear relationships between the input features and the target variable.2.2 Evolutionary AlgorithmsEvolutionary algorithms, such as genetic algorithms and particle swarm optimization, are widely used in optimization problems. These algorithms imitate the process of natural evolution and can effectively search for the optimal solution within a large parameter space. In stock prediction, evolutionary algorithms can optimize the neural network's parameters to enhance its performance.2.3 Partial Neural Evolution Network (P-NEN)Inspired by the above two techniques, we propose a novel model called P-NEN for stock prediction. P-NEN consists of a neural network architecture and an evolutionary algorithm. The neural network is responsible for capturing the patterns and relationships in the historical stock data, while the evolutionary algorithm optimizes the neural network's parameters. P-NEN incorporates partial connections between neurons to improve the network's efficiency and prevent overfitting.3. Experimental ResultsTo evaluate the performance of P-NEN, we conduct experiments on real-world stock data. We compare P-NEN with traditional neural networks, genetic algorithms, and other state-of-the-art models. The evaluation metricsinclude accuracy, precision, recall, and F1-score. The results demonstrate that P-NEN outperforms the baseline models and achieves higher accuracy in stock prediction.4. DiscussionThe experimental results validate the effectiveness of P-NEN in stock prediction. By combining neural networks and evolutionary algorithms, P-NEN takes advantage of both techniques and achieves improved predictive performance. The partial connections in P-NEN further enhance the model's efficiency and prevent overfitting. However, there are still challenges in stock prediction, such as data quality and forecasting market trends during extreme events. Future research should focus on addressing these challenges and further improving the accuracy and reliability of stock prediction models.5. ConclusionIn this study, we propose a new approach called Partial Neural Evolution Network (P-NEN) for stock prediction. P-NEN combines the strengths of neural networks and evolutionary algorithms to enhance the predictive performance. Through experiments and comparisons, we demonstrate the effectiveness of P-NEN in stock prediction. We believe that P-NEN has the potential to be applied in real-world financial scenarios and contribute to more accurate and reliable stock predictions.References:[1] Zhang, Y., Cheng, J., & Zhang, G. (2020). A neural network based method for stock prediction. Neurocomputing, 380, 173-184.[2] Li, S., Wu, Q., & Yang, G. (2019). Stock price prediction based on hybrid model of chaotic maps and quantum particle swarm optimization algorithm. Applied Soft Computing, 74, 653-662.[3] Wang, Y., & Ji, Y. (2018). Stock price prediction using deep neural network with dilated convolutional layers. Neurocomputing, 275, 187-197.。
科技英语试题及答案
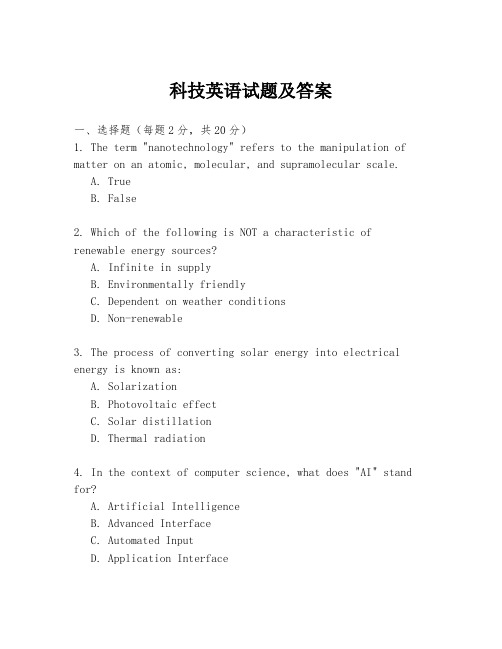
科技英语试题及答案一、选择题(每题2分,共20分)1. The term "nanotechnology" refers to the manipulation of matter on an atomic, molecular, and supramolecular scale.A. TrueB. False2. Which of the following is NOT a characteristic of renewable energy sources?A. Infinite in supplyB. Environmentally friendlyC. Dependent on weather conditionsD. Non-renewable3. The process of converting solar energy into electrical energy is known as:A. SolarizationB. Photovoltaic effectC. Solar distillationD. Thermal radiation4. In the context of computer science, what does "AI" stand for?A. Artificial IntelligenceB. Advanced InterfaceC. Automated InputD. Application Interface5. The term "genome" is associated with:A. The complete set of genes in an organismB. The structure of a cellC. The study of geneticsD. The process of cell division6. What is the primary function of a transistor in an electronic circuit?A. To amplify signalsB. To store dataC. To convert light into electricityD. To filter signals7. The "Internet of Things" (IoT) refers to:A. A network of interconnected devicesB. The global network of computersC. A collection of internet protocolsD. The study of internet security8. Which of the following is a type of biotechnology?A. Genetic engineeringB. Quantum computingC. NanolithographyD. Nuclear fusion9. The "Greenhouse Effect" is related to:A. The warming of the Earth's surfaceB. The cooling of the Earth's surfaceC. The process of photosynthesisD. The formation of the ozone layer10. What does "CRISPR" stand for in the field of molecular biology?A. Clustered Regularly Interspaced Short Palindromic RepeatsB. Computer-Aided Research in Scientific ProjectsC. Comprehensive Research in Innovative ScienceD. Computational Research in Systematic Processes二、填空题(每题1分,共10分)1. The unit of electrical resistance is the ______.2. The process of converting sound waves into electrical signals is known as ______.3. In physics, the term "entropy" is used to describe the level of ______ in a system.4. The study of the chemical composition of planets is known as ______.5. The term "cybersecurity" refers to the protection of______ from cyber threats.6. The process of converting electrical energy into light is known as ______.7. The smallest unit of life that can replicate itself is called a ______.8. The process of creating new substances from existing ones is known as ______.9. The study of the structure and function of cells is known as ______.10. The process of converting light energy into chemical energy is known as ______.三、简答题(每题5分,共30分)1. Explain the concept of "machine learning" in artificialintelligence.2. Describe the role of a semiconductor in modern electronics.3. What is the significance of biodiversity in the context of environmental science?4. Discuss the potential impact of nanotechnology on medicine.四、论述题(共40分)1. Discuss the ethical considerations involved in the development and use of genetic engineering technologies. (20分)2. Analyze the potential benefits and challenges of implementing a global Internet of Things (IoT) network. (20分)答案:一、选择题1. A2. D3. B4. A5. A6. A7. A8. A9. A10. A二、填空题1. ohm2. transduction3. disorder4. cosmochemistry5. information systems6. electroluminescence7. cell8. synthesis9. cytology10. photosynthesis三、简答题1. Machine learning is a subset of artificial intelligence that enables computers to learn from and make decisions based on data, improving at tasks over time through experience without being explicitly programmed.2. Semiconductors are materials with electrical conductivity between that of a conductor and an insulator. They arecrucial in electronic devices like transistors and diodes, allowing for the control of electrical current and the amplification of signals.3. Biodiversity is significant in environmental science as it ensures the stability of ecosystems, supports ecological processes, and provides a variety of services and resources that are vital for human survival and well-being.4. Nanotechnology has the potential to。
A system-level multiprocessor system-on-chip modeling framework

Kashif Virk Jan Madsen Informatics and Mathematical Modeling Technical University of Denmark {virkjan} @imm.dtu.dk
0-7803-8558-6/04/$20.00 02004 IEEE
81
the system-level in our modeling framework.
2 System-Level Modeling
To address the system-level design challenges described above, we need an extended system-on-chip design process, including the effects of the network-on-chip, with the ability to evaluate options and make critical architectural decisions based on a system-level representation in advance of a detailed design. A key pre-requisite is a library of ahstract component models that captures their respective performance, power, and physical characteristics. The primary goal of system-level modeling for emhedded systems is to formulate a model within which a broad class of designs can be developed and explored. Moreover, the difficulty of verifying the design of complex systems can be reduced by decomposing a system into smaller subsystems, independently verifying an implementation of the subsystems, and then proving that the composition of the subsystem specifications satisfies the overall system specification. In order to do so, accurate modelling of the sys.em and all the interrelationships among the diverse processors, software processes, physical interfaces and interconnections is needed. The scheduling problem, central to the analysis of the complexity of concurrent programs, depends on the way in which the scheduled tasks are mapped on the processing elements which, in tum, is linked with the physical architecture of the computing platforms. A real-time operating system is meant to provide some assurances ahout the timely performance of tasks. Unfortunately, most mechanisms used in the basic RTOS services are not compositional in nature. Even if a mechanism can provide assurances individually to each task, there is no systematic way to provide assurances for an aggregate of two except in trivial cases. To supporl the designers of single chip-based embedded systems, which includes multiprocessor platforms running dedicated RTOS’s, we have developed a modeling environment based on SystemC [2,4]. In our abstract RTOS modeling framework, we deal with generalized abstract tasks, processing elements, and communication infrastructures. For the purposes of modelling, three distinct but closely-related RTOS services have heen identified, namely, task scheduling, execution synchronization, and resource allocation.
英语作文-集成电路设计行业中的行业热点与前沿技术
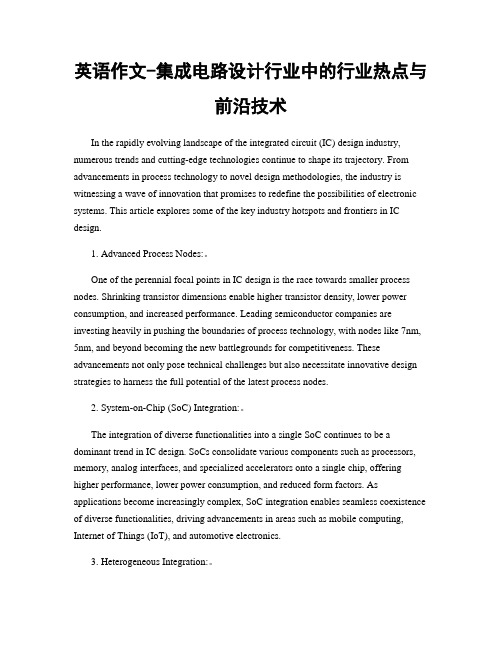
英语作文-集成电路设计行业中的行业热点与前沿技术In the rapidly evolving landscape of the integrated circuit (IC) design industry, numerous trends and cutting-edge technologies continue to shape its trajectory. From advancements in process technology to novel design methodologies, the industry is witnessing a wave of innovation that promises to redefine the possibilities of electronic systems. This article explores some of the key industry hotspots and frontiers in IC design.1. Advanced Process Nodes:。
One of the perennial focal points in IC design is the race towards smaller process nodes. Shrinking transistor dimensions enable higher transistor density, lower power consumption, and increased performance. Leading semiconductor companies are investing heavily in pushing the boundaries of process technology, with nodes like 7nm, 5nm, and beyond becoming the new battlegrounds for competitiveness. These advancements not only pose technical challenges but also necessitate innovative design strategies to harness the full potential of the latest process nodes.2. System-on-Chip (SoC) Integration:。
Role of the Metal-Oxide Support in the Catalytic Activity
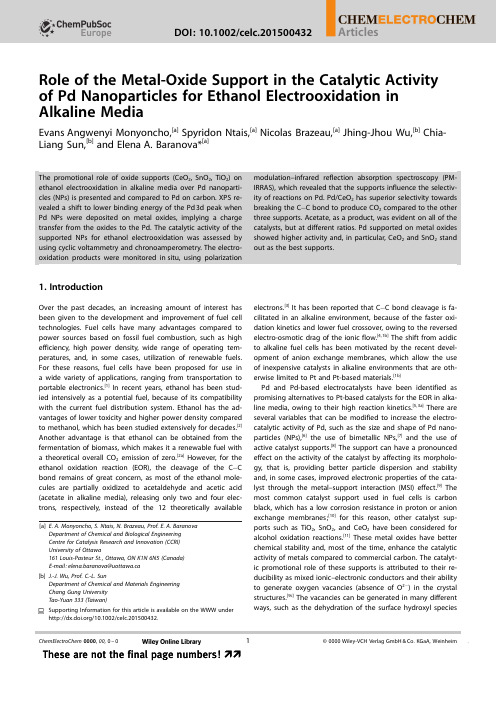
Role of the Metal-Oxide Support in the Catalytic Activity of Pd Nanoparticles for Ethanol Electrooxidation in Alkaline MediaEvans Angwenyi Monyoncho,[a]Spyridon Ntais,[a]Nicolas Brazeau,[a]Jhing-Jhou Wu,[b]Chia-Liang Sun,[b]and Elena A.Baranova*[a]1.IntroductionOver the past decades,an increasing amount of interest has been given to the development and improvement of fuel cell technologies.Fuel cells have many advantages compared to power sources based on fossil fuel combustion,such as high efficiency,high power density,wide range of operating tem-peratures,and,in some cases,utilization of renewable fuels.For these reasons,fuel cells have been proposed for use in a wide variety of applications,ranging from transportation to portable electronics.[1]In recent years,ethanol has been stud-ied intensively as a potential fuel,because of its compatibility with the current fuel distribution system.Ethanol has the ad-vantages of lower toxicity and higher power density compared to methanol,which has been studied extensively for decades.[2]Another advantage is that ethanol can be obtained from the fermentation of biomass,which makes it a renewable fuel with a theoretical overall CO 2emission of zero.[2a]However,for the ethanol oxidation reaction (EOR),the cleavage of the C ÀC bond remains of great concern,as most of the ethanol mole-cules are partially oxidized to acetaldehyde and acetic acid (acetate in alkaline media),releasing only two and four elec-trons,respectively,instead of the 12theoretically availableelectrons.[3]It has been reported that C ÀC bond cleavage is fa-cilitated in an alkaline environment,because of the faster oxi-dation kinetics and lower fuel crossover,owing to the reversed electro-osmotic drag of the ionic flow.[4,1b]The shift from acidic to alkaline fuel cells has been motivated by the recent devel-opment of anion exchange membranes,which allow the use of inexpensive catalysts in alkaline environments that are oth-erwise limited to Pt and Pt-based materials.[1b]Pd and Pd-based electrocatalysts have been identified as promising alternatives to Pt-based catalysts for the EOR in alka-line media,owing to their high reaction kinetics.[5,3a]There are several variables that can be modified to increase the electro-catalytic activity of Pd,such as the size and shape of Pd nano-particles (NPs),[6]the use of bimetallic NPs,[7]and the use of active catalyst supports.[8]The support can have a pronounced effect on the activity of the catalyst by affecting its morpholo-gy,that is,providing better particle dispersion and stability and,in some cases,improved electronic properties of the cata-lyst through the metal–support interaction (MSI)effect.[9]The most common catalyst support used in fuel cells is carbon black,which has a low corrosion resistance in proton or anion exchange membranes;[10]for this reason,other catalyst sup-ports such as TiO 2,SnO 2,and CeO 2have been considered for alcohol oxidation reactions.[11]These metal oxides have better chemical stability and,most of the time,enhance the catalytic activity of metals compared to commercial carbon.The catalyt-ic promotional role of these supports is attributed to their re-ducibility as mixed ionic–electronic conductors and their ability to generate oxygen vacancies (absence of O 2À)in the crystal structures.[9c]The vacancies can be generated in many different ways,such as the dehydration of the surface hydroxylspecies[a]E.A.Monyoncho,S.Ntais,N.Brazeau,Prof.E.A.BaranovaDepartment of Chemical and Biological Engineering Centre for Catalysis Research and Innovation (CCRI)University of Ottawa161Louis-Pasteur St.,Ottawa,ON K1N 6N5(Canada)E-mail:elena.baranova@uottawa.ca [b]J.-J.Wu,Prof.C.-L.SunDepartment of Chemical and Materials Engineering Chang Gung University Tao-Yuan 333(Taiwan)Supporting Information for this article is available on the WWW under /10.1002/celc.201500432.ArticlesDOI:10.1002/celc.201500432(OHÀ)and the reduction of accessible metal cations in the oxides through chemical means.The catalytic promotional properties of oxide supports in electrocatalysis have attracted the interest of many researchers, for instance,TiO2,[11e,g–i,12]SnO2,[3b,11a,c,m,n]and CeO2[11b,j,k]have been extensively investigated.Focusing on Pd-based catalysts on these supports for ethanol electrooxidation,Hu et al.pre-pared Pd NPs on carbonized TiO2nanotubes for ethanol elec-trooxidation in alkaline media,and reported that the electroca-talyst with a1:1mass ratio of Pd to TiO2/C for Pd/TiO2/C gave the best performance compared to Pd/C and Pd/TiO2.[11i]Mao et ed the impregnation reduction method to prepare carbon-supported PdSn/SnO2and showed that it had a higher current density for ethanol electrooxidation in alkaline media compared to Pd/C,SnO2/C,and PdSn/C.[11n]They showed that SnO2improved Pd particle distribution.Bambagioni et al.have shown that the addition of CeO2as a co-support to carbon for Pd NPs(Pd/C/CeO2)improved the power density of the direct alkaline ethanol fuel cell by a factor of two,as compared to Pd/C.[11b]Uhm et al.synthesized well-ordered arrays of free-standing Pd-CeO2nanobundles and reported that the catalysts had an increased number of oxygen species on the surface,re-sulting in a significant increase in their catalytic activity for the EOR in KOH.[11j]Shen and co-workers conducted a comparative study of ethanol electrooxidation on Pt/C and Pd/C catalysts, promoted by CeO2in alkaline media,and reported that CeO2 significantly improved the catalyst activity and poison toler-ance.[13]Although the promotional effect of these oxide sup-ports is evident in these studies,no direct comparison for eth-anol electrooxidation reaction on Pd NPs supported on TiO2, CeO2,SnO2,and C exists.Furthermore,there are several other questions regarding the role of the support,such as the sup-ports influence on NP size and elemental surface composition (i.e.surface oxides);in addition,the catalytic activity of Pd still remains to be elucidated.In this work,we conduct a comparative study of Pd NPs sup-ported on TiO2,SnO2,CeO2,and a conventional carbon sup-port,where the Pd NPs are prepared using the same synthesis procedure and the same metal loading.This approach allows us to evaluate the role/effect of the support on a number of properties of Pd NPs:1)the influence of the support on the particle and crystallite sizes,using transmission electron mi-croscopy(TEM)and X-ray diffraction(XRD)data;2)the effect of the support on the surface composition(oxidation states of Pd)and electronic effect,using X-ray photoelectron spectros-copy(XPS)data;3)the effect of support on the chemisorption and dispersion properties through CO stripping data;and 4)the effect of the support on the electrocatalytic properties of Pd NPs for ethanol oxidation in alkaline media by using cyclic voltammetry(CV),chronoamperometry(CA),and polari-zation modulation–infrared reflection absorption spectroscopy (PM-IRRAS)data.To this end,Pd NPs supported on CeO2,SnO2, TiO2,and carbon were prepared using sodium borohydride as a reducing agent in an aqueous medium.The EOR was studied in1m(KOH+C2H5OH)using CV and CA,and the CA was cou-pled with the in situ identification of products using PM-IRRAS.A discussion is provided to correlate the first three properties (1–3)to the electrocatalytic activity of Pd NPs induced by metal–support interactions.2.Results and Discussion2.1.Physicochemical Characterization of Supported Pd NPs The morphology and NP size distribution of the four catalysts were determined by using TEM,and the resulting microscopy images and histograms are shown in Figure1.The microscopy images show that Pd NPs were relatively well dispersed in all four of the supports tested with some degree of agglomeration,which is more evident on the SnO2 support.The histograms show the particle-size distribution of the Pd NPs for each catalyst synthesized.The average sizes of the NPs were10.4,12.3,12.6,and14.2nm for Pd NPs on SnO2, CeO2,C,and TiO2,respectively.It is interesting to note that the Pd NPs had the smallest size on SnO2and the largest size on the TiO2support,whereas the size was comparable between C and CeO2.The small specific surface area of TiO2(Table1)may be responsible for the larger size of the Pd clusters.The crystal structure of the NPs was determined by using XRD patterns,as presented in Figure2.The diffraction pattern shows that the Pd NPs retained in the bulk face-centered cubic (fcc)structure;the*symbol shows the signature peaks forfcc Figure1.TEM images of Pd NPs supported on a)CeO2,b)carbon,c)SnO2, and d)TiO2.The corresponding histograms on the right show the NP size distribution.structures.The signature fcc peaks were detected at 40.08,46.5,and 68.28on the 2q scale for all of the samples,which correspond to the (111),(200),and (220)planes,respectively.All extra peaks on the diffraction patterns correspond to the respective oxide support,as shown in the diffraction patterns of the pure supports in Figure S1in the Supporting Informa-tion.The crystallite size of the Pd NPs was estimated by using the Scherrer equation,which yields the crystallite size of the NPs.[14]The crystallite size was estimated by using the Pd(111)peak,which was confirmed to have no significant overlap with the support peaks,as shown in Figure S1.The crystallite sizes of the particles were calculated to be 10.8,7.5,9.5,and 15.2nm for Pd NPs supported on C,SnO 2,CeO 2,and TiO 2,re-spectively.The crystallite size trends are in a good agreement with the particle-size diameters found from TEM images,as shown in Table 1.The lower crystallite values compared to par-ticle sizes for Pd/CeO 2and Pd/SnO 2indicate the agglomeration of crystals in those supports.Therefore,the broader Pd peaks for CeO 2and SnO 2(Figure 2)provide evidence for the smaller crystallite sizes of the NPs,but these agglomerate together to form the larger grains detected by TEM.[15]The surface composition of the supported Pd NPs on the dif-ferent oxide supports was determined by XPS.Figure 3shows the high-resolution Pd 3d XPS peaks for all four samples.Table 2summarizes the peak positions,the full width at halfmaximum (FWHM),the atomic percentage of each component,and the chemical environment assignment of the peaks.The deconvolution of the Pd 3d peaks reveals the existence of Pd only in the metallic state for the SnO 2and TiO 2supports.How-ever,in the case of NPs supported on carbon and CeO 2,the deconvolution reveals the existence of Pd atoms that are also in higher oxidation states.In the case of Pd/C,the deconvolu-tion revealed the existence of four peaks at 335.4,336.4,337.5,and 338.4eV that are attributed to metallic Pd,PdO,PdO 2,and PdCl x ,respectively.[16]In the case of Pd/CeO 2,these deconvolut-ed components can be found at 334.6,336.1,337.4,and 338.4eV,respectively.The existence of the peak at 338.4eV and its assignment to PdCl x species is further supported by the detected Cl 2p peaks (not shown here)for the Pd/C and Pd/CeO 2catalysts.By using the intensities of the Cl 2p and of the corresponding Pd 3d component,it was found that the Cl/Pd atomic ratio is 1.2andFigure 2.XRD patterns of supported Pd NPs on various supports as shown.The symbol (*)corresponds to the fcc structure diffractions forPd.1.35for the carbon and ceria supported catalysts,respectively.The existence of PdCl x species on the surface of Pd prepared by chlorinated precursors has been reported before,[17]and it seems that their presence can be affected by the nature of the support.The removal of chlorine can take place by a drying–reduction pretreatment,but still a small amount may remain in the catalyst.[17b]Our XPS results show that for Pd NPs supported on oxides (CeO 2,SnO 2,and TiO 2),the peak attributed to the metallic state shows a shift to lower binding energies compared to the corre-sponding peak in the case of carbon-supported NPs (Pd/C).For Pd/SnO 2,the peak was detected at 335.2eV,whereas in the case of Pd/TiO 2and Pd/CeO 2,the metallic state (Pd 0)peak is shifted to lower binding energies by 0.6and 0.8eV,respective-ly.Similar shifts of the Pd 3d peak have been reported before for Pd supported on these oxides.[18]The observed shift of thePd 3d peaks to lower binding energies for Pd NPs supported on oxides is attributed to a metal–support interaction,where the charge is transferred from the support to Pd NPs.[19]Upon the contact of two metal atoms with different electronegativi-ties,the charge will be transferred from the atom with the lower electronegativity to the atom with higher electronegativ-ity until the energy level of the electrons at the interface is equilibrated.The electronegativities for the atoms involved here are,in increasing order,1.12,1.54,1.96,2.20,and 2.55for Ce,Ti,Sn,Pd,and C,respectively.[20]It is interesting to note that Pd was 100%reduced on SnO 2and TiO 2but only 67%re-duced on CeO 2,as shown by the XPS data in Table 2,which would seem to contradict the electronegativity difference trends.However,the observed difference could be explained based on the crystal structure of the supports.CeO 2has a fluo-rite-type structure,whereas SnO 2and TiO 2have rutile-type crystal structures.First,it is important to mention that the shift of the metallic peak follows the electronegativity trend as ex-pected,that is,the largest shift occurs for Pd NPs supported on CeO 2because of its lowest electronegativity value,and vice versa to NPs on SnO 2.It follows then that the structure of the supports would be responsible for the lower percentage (67%)reduction of Pd atoms on CeO 2,because the samples were prepared by using the same protocol and conditions.It is well known that CeO 2,owing to its non-stoichiometry,has the abili-ty to undergo conversion between Ce 4+and Ce 3+quite easily,[21]which can explain the presence of Pd oxides in the Pd/CeO 2catalyst.Figure 4presents the XPS spectra of Ce 3d for Pd/CeO 2(Fig-ure 4a),Ti 2p for Pd/TiO 2(Figure 4b),and Sn 3d for Pd/SnO 2(Figure 4c)with characteristic peaks of the supports.The Ce 3d XPS spectrum is rather complex,owing to the electron correla-tion phenomena.In general,six peaks are characteristic of Ce 4+,whereas four are characteristic of Ce 3+.The Ce 3d 5/2peaks at 882,888.7,and 897.8eV and their corresponding Ce 3d 3/2components at 900.7,906.9,and 916.1eV (dotted lines on Figure 4a)are attributed to cerium atoms in CeO 2.The Ce 3d 5/2peaks at 880.3and at 899.1eV with their correspond-ing 3d 3/2lines at 885.8and 903.5eV (dashed lines on Fig-ure 4a)reveal the existence of cerium atoms in the Ce 3+oxida-tion state and,more specifically,in Ce 2O 3.[22]For the other two samples,Ti 2p 3/2and Sn 3d 5/2peaks are detected at 458.4and 486.8eV,respectively,and are characteristic of Ti and Sn atoms in the 4+oxidation state.[23]Although,the relatively large FWHM of the two peaks (ca.1.5eV)implies the existence of Ti and Sn atoms in more than one chemical environment,but more studies are necessary to confirm this.2.2.Electrochemical Measurements 2.2.1.CO StrippingThe CO stripping charge was used to determine the electro-chemically active surface area (ECSA)of the four catalysts by using the protocol reported in the literature.[24]Figure S2shows the first and second cycle of the CO stripping CV curves for the four catalysts.The area between the two cyclesfrom Figure 3.Pd 3d XPS peak of Pd supported on a)CeO 2,b)TiO 2,c)SnO 2,and d)carbon.The shifting of binding energy for Pd 0(335.4eV,vertical line)to lower values indicates the level of Pd–MO 2(M =Sn,Ti,and Ce)interactions.the potential of À0.17to 0.15V and monolayer stripping charge of 420m C cm À2were used to determine the ECSA.[24]The ECSA values are shown in Table 1.The oxidation peak po-tential for CO was found to be À0.11V for all samples.Howev-er,there is significant charge distribution depending on the support,which indicates the differences in NP dispersion and CO binding on the surfaces.Pd NPs on SnO 2were found to be the smallest (crystallite size =7.5nm,particle size =10.4nm),hence leading to the highest ECSA of 0.92cm 2.Interestingly,this predicted value (0.93cm 2)is the same as the real surface area of a polycrystalline Pd electrodes [taking the average of the experimental values for (111),(100),and (110)surfaces]after CO stripping on Pd in an electrochemical environment.[25]Note that Pd was 100%metallic on SnO 2and TiO 2,so it is not surprising to have such perfect polycrystalline NPs.However,one may wonder why we do not have such a similar real sur-face area for Pd NPs on TiO 2.First,Pd NPs on TiO 2are the larg-est (crystallite size =15.2nm,particle size =14.2nm).Second,TiO 2is a poor conductor that will insulate some parts of the NPs.The Pd NPs supported on CeO 2and C have similar ECSAvalues,but they are lower than that of Pd/SnO 2,which is con-sistent with the fact that they have approximately same per-centage of metallic Pd,based on XPS data in Table 2.2.2.2.Ethanol ElectrooxidationFirst,we present the CV curves of the NPs in 1m KOH,and then CV curves in 1m (KOH +C 2H 5OH)solution.The character-istic CV curves of the four catalysts in 1m KOH at a scan rate of 25mV s À1are shown in Figure 5.The voltammograms show similar features but with different current densities,owing to variations between the NP–support interactions that,in turn,alter the catalytic activity at the interfaces.In the anodic scan,there are peaks below À0.4V (labelled I in Figure 5),which are attributed to the oxidation of adsorbed and absorbed hydrogen on Pd NPs.The anodic peaks labelled II,observed between À0.35and À0.25V,are generally attribut-ed to the adsorption of hydroxyl groups on the surface of Pd.[26,6a]The peaks labelled III,situated at potentials higher than À0.25V,correspond to the transformation of adsorbed hydroxyl groups to higher valence oxides (PdO x ),following re-action pathways in Equations (1)–(3):[3a]Pd þOH À!Pd ÀOH ads þe Àð1ÞPd ÀOH ads þPd-OH ads !Pd ÀO ads þH 2O ð2ÞPd ÀOH ads þOH À!Pd ÀO ads þH 2O þe Àð3ÞIn the cathodic scan,the peaks labelled IV,with a minimum situated at ~À0.15V (except for Pd/SnO 2at ca.À0.25V),are attributed to the reduction of the PdO x species formed during the anodic scan.The shoulders seen on peak IV for Pd/CeO 2can be attributed to the reduction of different oxidation states of Pd atoms on various Pd crystalline planes and on low coor-dination sites.For Pd/SnO 2,reduction peak IV is broad and shifted to lower potentials,indicating that the reductionpro-Figure 4.XPS peak of Ce 3d for Pd/CeO 2(A),Ti 2p for Pd/TiO 2(B),and Sn 3d for Pd/SnO 2(C).Figure 5.Cyclic voltammograms of Pd NPs deposited on carbon,CeO 2,SnO 2,and TiO 2in 1m KOH at v =25mV s À1.The vertical lines show the potentials in which the CA experiments and in situ monitoring of products by PM-IRRAS were conducted during ethanol electrooxidation (vide infra).cess is thermodynamically unfavorable.When the applied po-tential is lower than À0.45V,the cathodic current decreases,owing to a combination of three overlapping phenomena:1)the adsorption of H on the Pd surface;2)the diffusion of H into the lattices of Pd,allowing more H atoms to be adsorbed on the surface;and 3)the evolution of H 2starts to take place as the potential lowered further.The CV curves for ethanol electrooxidation over the four cat-alysts in 1m (KOH +C 2H 5OH)are shown in Figure 6.The vol-tammograms were collected by scanning the potential from an initial open-circuit voltage towards the anodic direction to a maximum potential of 0.15V,and then in the cathodic direc-tion to a minimum potential of À0.65V at a rate of 5mV s À1.The graphs showing the details of this protocol,that is,the first two cycles of each catalyst,are provided in the Supporting Information (Figure S3).In Figure S3,the charge transfer (Q )in both the anodic and cathodic sweeps is readily available as the area under the current peaks.The open-circuit potential (OCP)of four catalysts was found from Figure S3and the value increases in the order of Pd/TiO 2<Pd/C <Pd/SnO 2<Pd/CeO 2with values of À0.43,À0.52,À0.57,and À0.62V,respectively.The CV curves for the supports in 1m (KOH +C 2H 5OH)are provided in the Supporting Information (Figure S4),and con-firm that the supports have no catalytic activity for ethanol electrooxidation in alkaline media.Figure 7compares the linear-sweep voltammetry of the four catalysts in 1m (KOH +C 2H 5OH).The figure shows that,for Pd NPs supported on TiO 2and C,the onset potential is around À0.33V,whereas for NPs supported on SnO 2and CeO 2it is shifted to a lower value of À0.45V.The trend of the anodic current magnitude is consistent with that of the ECSA and maximum anodic current densities (I a,max )of the catalyst.At I a,max ,the catalyst is deactivated,owing to the formation of sur-face oxides and adsorbed oxidized species.Therefore,it is in-teresting to see the role of the supports in influencing the po-tential at which this phenomenon occurs.It was found that Pd NPs on the C support are easily deactivated at a potential of À0.04V (2.97mA cm À2),and those on SnO 2and TiO 2are deacti-vated at À0.02V (5.86and 1.63mA cm À2,respectively).But,the NPs on CeO 2were very resistant,deactivating at 0.01V (4.92mA cm À2).This observation indicates that CeO 2is capable to accommodating more surface oxides (O 2À)than the other supports (vide infra).During the reverse scan (Figure 6),the potential of the work-ing electrode is gradually lowered,allowing the transfer of electrons into the catalysts (reduction process).During the re-verse scan,a positive current was obtained,starting at around À0.05V and rapidly increasing (unlike the gradual increase in anodic scan)to a maximum current (I r,max ),which depends on the NP support,and then gradually decaying to zero at lower potentials.The differences observed in the reverse current peak shapes (see Figure 6)are related to the ability of the oxi-dized species to diffuse into the bulk solution,hence allowing fresh ethanol to access the catalyst surface.For instance,Pd/CeO 2showed a sharp current increase at À0.09V during the backward scan,which can be explained by the known CeO 2phenomenon,that is,it releases lattice oxygen (O 2À)when re-duced.[27]Therefore,the released oxygen species could help to push oxidized intermediates from the surface,hence allowing rapid access of the fresh species for oxidation.As can be seen,CeO 2and SnO 2stand out as the best performers.CeO 2is of particular interest,based on the cyclic voltammograms in Figure 6a.2.3.In Situ Identification of Ethanol Electrooxidation ProductsThe CA technique coupled with PM-IRRAS was used to investi-gate the ethanol electrooxidation products.PM-IRRAS allows us to distinguish between the oxidation species on the elec-trode surface (the difference between p-and s-polarized reflec-tion absorption spectra)and the oxidation species in the bulk electrolyte within the thin cavity between the CaF 2window and the electrode (the average of the p-and s-polarized reflec-tion absorption spectra)at each potential.Therefore,the spec-tra for the species on the electrode surface and the spectraof Figure 6.Cyclic voltammograms of Pd NPs supported on CeO 2(a),SnO 2(b),TiO 2(c),and carbon (d)in 1m (KOH +C 2H 5OH)at n =5mV s À1.The current densities are given per the ECSA determined through the CO strippingmethod.Figure 7.Linear-sweep voltammetry of the four catalysts in 1m (KOH +C 2H 5OH)at v =5mV s À1.the average species in the thin cavity will be labeled as “sur-face”and “bulk”,respectively.The PM-IRRAS spectra for ethanol electrooxidation products are shown in Figure 8.The left and right figures show the sur-face and bulk species,respectively,for the four catalysts after holding potential at À0.3V for 10min.Additional spectra to show how the products evolved with time are shown in Fig-ure S5.The presence of peaks at 1560and 1423cm À1in the surface and bulk spectra are evidence for the acetate (CH 3COO À)produced in the four catalysts.However,the amount of acetate varied from one support to another,where Pd NPs supported on TiO 2were the least active,whereas the Pd NPs on SnO 2were the most active.Further spectra of the most active catalysts were collected at lower potentials close to the onset potential for EOR at À0.5and À0.4V for Pd/CeO 2and Pd/SnO 2,respectively,and are shown in Figure 9.The C ÀC bond corresponds to the CO 2peak at 2345cm À1.The spectra demonstrate that the oxide supports have a sig-nificant influence on the selectivity of the electrooxidation spe-cies,in particular those species with absorption peaks centered at 1724,1919,2345,and 2700cm À1.Of key interest is that the selectivity towards breaking Pd/CeO 2showed superior selectiv-ity in breaking the C ÀC bond.In this regard,the utility of PM-IRRAS came into play in distinguishing that the produced CO 2desorbs/diffuses from the surface into the bulk.Desorption ofCO 2into the bulk is confirmed by the higher intensity of the peak at 2345cm À1compared to that of the surface,as shown in Figures 9a and 9b.With increasing time and potential,the Figure 8.PM-IRRAS spectra generated during ethanol electrooxidation on Pd NPs supported on,SnO 2,CeO 2,C,and TiO 2after holding potential at À0.3V versus Hg/HgO for 10min in 1m (KOH +C 2H 5OH).The top and bottom shows the bulk species and surface species,respectively.Figure 9.PM-IRRAS spectra generated during ethanol electrooxidation on Pd/CeO 2at À0.5V at increments of 5min (upper panel)and on Pd/SnO 2at À0.4V at increments of 5min (lower panel)in 1m (KOH +C 2H 5OH).Panels (a)and (c)refer to surface species,whereas (b)and (d)show bulk species.competition increases between breaking the C ÀC bond and forming the acetate and other species at 1724cm À1for the Pd/CeO 2NPs.The origin of the species absorbing at 1724,1919,and 2700cm À1is currently being investigated.Pd/SnO 2did not show any evidence of breaking the C ÀC bond;instead,it showed high selectivity and high reaction kinetics towards the production of the species absorbing at 2700cm À1.Even at low potential (À0.4V),acetate and the species at 2700cm À1were the main products,as shown Figures 9c and 9d.This ob-servation highlights the promotional catalytic effect of metal-oxide supports compared to the commonly used carbon support.It is important to note the difference between the spectra of the surface and bulk species between 1600and 2000cm À1.The bulk spectra have a broad peak centered at 1724cm À1,which is well shaped for Pd/CeO 2,but it is missing on the sur-face spectra.Similarly,the surface spectra exhibit a broad peak around 1919cm À1,which is well pronounced on Pd/SnO 2.The peak at 1724cm À1maybe attributed to the C =O vibrations from acetaldehyde (CH 3CHO)or the aldol product [CH 3CH(OH)CH 2CHO]formed from the desorbed acetaldehyde,but it is subject to further investigation.This suggestion is based on the fact that,on the surface,the carbonyl double-bond character is absent,because of the molecules interacting with the surface and owing to its polarization from the applied potential.The CA responses of ethanol oxidation over the four Pd cat-alysts recorded at À0.40,À0.2,À0.15,and À0.09V are shown in Figure 10.The current densities of Pd/TiO 2and Pd/C aremuch lower compared to Pd/CeO 2and Pd/SnO 2.In general,the Pd NPs supported on metal oxides have a higher activity for ethanol electrooxidation compared to the Pd/C catalyst,which is consistent with the amount of acetate produced.The significant difference in the current densities obtained implies that the reaction kinetics at the interface is different on each support,in agreement with the reaction selectivity demon-strated by the PM-IRRAS spectra.The CA curves reveals further important features of the reac-tion kinetics at the electrolyte–NP interface based on the cur-rent density traces.For Pd NPs on CeO 2and SnO 2,the currents showed increasing trends as soon as the potential was changed/stepped,and then decreased gradually.The initial in-creasing current densities (Figure 10)show that the NPs on CeO 2and SnO 2are very active,in agreement with CV and PM-IRRAS results.The high catalytic activity of Pd NPs on ceria and tin oxide could be correlated to metal–support interaction (MSI)gener-ated between the Pd and the oxides;however,the particle-size effect could also play a role.The smaller the NPs,the larger the active surface area that is available for the reaction as well as a stronger MSI,because of the shorter charge-trans-fer distances between the two solids.A clear trend is observed between the crystallite sizes (Table 1)and the current densities (Figures 7and 10).The smaller the crystallite size,the higher the current density obtained,which explains the higher cata-lytic activity for Pd/SnO 2and Pd/CeO 2.The poor performance of Pd/TiO 2would be attributed to their large crystallite/particle sizes and perhaps the weaker MSI.The origin of the MSI in Pd NPs supported on oxides could be attributed to:1)the higher availability of hydroxyl ions or oxygenated species (O d À)from the support lattice,which accel-erates the oxidation rate of adsorbed ethanol intermediates es-pecially on CeO 2,and SnO 2;2)the change in the so-called Volta potential difference [27,28]between the support and Pd NPs,which will alter the Fermi level of electrons in Pd,leading to the modification of its catalytic properties.The Volta poten-tial difference between Pd and carbon black is 0.23eV and is the lowest among all of the catalysts investigated in the pres-ent work.The values for Pd/SnO 2,Pd/CeO 2,and Pd/TiO 2are 0.37,0.43,and 0.92eV,respectively;[29]however,the catalytic activity of Pd for the EOR does not follow the same trend,which indicates that Pd/TiO 2catalysts with the larger particle size and presence of Pd agglomerates negates the expected support effect.According to XPS measurements,the Pd 3d peak position is shifted to lower binding energies,confirming a charge transfer from the oxide support to Pd,which indi-cates that the MSI between the Pd and the metal-oxide sup-port contributes to the enhanced EOR.3.ConclusionsThe results presented in this study showed that the metal-oxide supports have a promoting effect on the electrocatalytic activity of Pd NPs for the EOR in alkaline media.A simple syn-thesis method was used to prepare Pd NPs,which were depos-ited in situ on the surface of different reducible metal oxides (CeO 2,TiO 2and SnO 2)and on a conventional carbon support.XRD showed that the synthesized Pd NPs have an fcc structure,similar to bulk Pd.The XPS spectra revealed a shift of the Pd 3d peaks to lower binding energies for Pd NPs deposited on oxides,owing to charge transfer from the oxide support,which results in a higher electron density on the NPs.The PM-IRRAS spectra demonstrated the influence of the support on the selectivity of the EOR on Pd NPs.Pd/CeO 2NPs showed superior selectivity for breaking the C ÀC bond,where-as Pd/SnO 2did not show any evidence of breaking the C ÀC Figure 10.Chronoamperograms of Pd on different supports in 1m (KOH +C 2H 5OH)at various applied potentials.The current densities are given per the ECSA determined through the CO stripping method。
Network_on_chip

Parameters Which determines the Network Performance
• Network Diameter: Maximum number of intermediate nodes between a source and any destination pair. • Connectivity: Number of direct neighbors of any switch node in the network. • Bandwidth: Measure of maximum rate (bits/second) of information flow in the network. • Latency is the time taken by a message to travel from the source to the destination.
KIRAN.V Asst.Professor.RVCE,Bangalore-59
KIRAN.V Asst.Professor.RVCE,Bangalore-59
KIRAN.V Asst.Professor.RVCE,Bangalore-59
NETWORK TOPOLOGY
• The topology of an NoC specifies the physical organization of the interconnection network • It defines how nodes, switches, and links are connected to each other • Classification Direct networks Indirect networks Irregular networks.
Symphony Enterprise Management和控制系统的Cnet高速数据通信网络说明
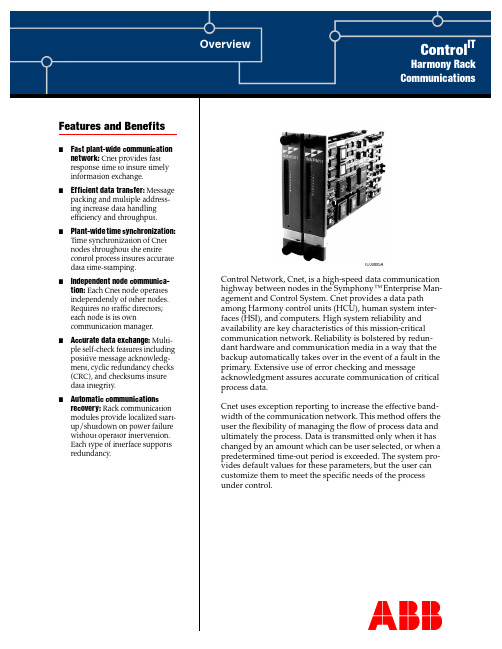
Features and Benefits Overview Control ITHarmony RackCommunications Control Network, Cnet, is a high-speed data communicationhighway between nodes in the Symphony™ Enterprise Man-agement and Control System. Cnet provides a data pathamong Harmony control units (HCU), human system inter-faces (HSI), and computers. High system reliability andavailability are key characteristics of this mission-criticalcommunication network. Reliability is bolstered by redun-dant hardware and communication media in a way that thebackup automatically takes over in the event of a fault in theprimary. Extensive use of error checking and messageacknowledgment assures accurate communication of criticalprocess data.Cnet uses exception reporting to increase the effective band-width of the communication network. This method offers theuser the flexibility of managing the flow of process data andultimately the process. Data is transmitted only when it haschanged by an amount which can be user selected, or when apredetermined time-out period is exceeded. The system pro-vides default values for these parameters, but the user cancustomize them to meet the specific needs of the processunder control.TC00895A■Fast plant-wide communication network: Cnet provides fastresponse time to insure timelyinformation exchange.■Efficient data transfer: Message packing and multiple address-ing increase data handlingefficiency and throughput.■Plant-wide time synchronization: Time synchronization of Cnetnodes throughout the entirecontrol process insures accuratedata time-stamping.■Independent node communica-tion: Each Cnet node operatesindependently of other nodes.Requires no traffic directors;each node is its owncommunication manager.■Accurate data exchange: Multi-ple self-check features including positive message acknowledg-ment, cyclic redundancy checks(CRC), and checksums insuredata integrity.■Automatic communications recovery: Rack communicationmodules provide localized start-up/shutdown on power failurewithout operator intervention.Each type of interface supportsredundancy.Harmony Rack CommunicationsOverviewHarmony rack communications encompasses various communication interfaces as shown inFigure1: Cnet-to-Cnet communication, Cnet-to-HCU communication, and Cnet-to-computercommunication.Figure 1. Harmony Rack Communications ArchitectureThe communication interface units transfer exception reports and system data, control, and con-figuration messages over Cnet. Exception reported data appears as dynamic values, alarms, and state changes on displays and in reports generated by human system interfaces and other system nodes. Exception reporting is automatic at the Harmony controller level. Specifically, the control-ler generates an exception report periodically to update data, after a process point reaches adefined alarm limit or changes state, or after a significant change in value occurs.Harmony Rack Communications Control NetworkCnet is a unidirectional, high speed serial data network that operates at a 10-megahertz or two-megahertz communication rate. It supports a central network with up to 250 system node connec-tions. Multiple satellite networks can link to the central network. Each satellite network supports up to 250 system node connections. Interfacing a maximum number of satellite networks gives a system capacity of over 62,000 nodes.On the central network, a node can be a bridge to a satellite network, a Harmony control unit, a human system interface, or a computer, each connected through a Cnet communication interface.On a satellite network, a node can be a bridge to the central network, a Harmony control unit, a human system interface, or a computer.Harmony Control UnitThe Harmony control unit is the fundamental control node of the Symphony system. It connects to Cnet through a Cnet-to-HCU interface. The HCU cabinet contains the Harmony controllers and input/output devices. The actual process control and management takes place at this level. HCU connection to Cnet enables Harmony controllers to:■Communicate field input values and states for process monitoring and control.■Communicate configuration parameters that determine the operation of functions such asalarming, trending, and logging on a human system interface.■Receive control instructions from a human system interface to adjust process field outputs.■Provide feedback to plant personnel of actual output changes.Human System InterfaceA human system interface such as a Signature Series workstation running Maestro or ConductorSeries software provides the ability to monitor and control plant operations from a single point. It connects to Cnet through a Cnet-to-computer interface. The number of workstations in a Sym-phony system varies and depends on the overall control plan and size of a plant. The workstation connection to Cnet gives plant personnel access to dynamic plant-wide process information, and enables monitoring, tuning, and control of an entire plant process from workstation color graphics displays and a pushbutton keyboard.ComputerA computer can access Cnet for data acquisition, system configuration, and process control. It con-nects to Cnet through a Cnet-to-computer interface. The computer connection to Cnet enablesplant personnel, for example, to develop and maintain control configurations, manage the system database, and create HSI displays remotely using Composer™engineering tools. There are addi-tional Composer and Performer series tools and applications that can access plant informationthrough a Cnet-to-computer interface.Cnet-to-Cnet Communication InterfaceThe Cnet-to-Cnet interfaces are the INIIR01 Remote Interface and the INIIL02 Local Interface.Figure2 shows the remote interface and Figure 3 shows the local interface.Harmony Rack CommunicationsFigure 2. Cnet-to-Cnet Remote Interface (INIIR01)Figure 3. Cnet-to-Cnet Local Interface (INIIL02)Harmony Rack Communications INIIR01 Remote InterfaceThe INIIR01 Remote Interface consists of the INNIS01 Network Interface Module and the INIIT12 Remote Transfer Module (Fig.2). This interface is a node on a central network that can communi-cate to an interface node on a remote satellite network. In this arrangement, two interfaces arerequired: one for the central network, and the other for the satellite network. Bidirectional commu-nication from the central network to the remote satellite network is through standard RS-232-Cports.The remote interface supports hardware redundancy. Redundancy requires a full set of duplicate modules (two INNIS01 modules and two INIIT12 modules on each network). The secondaryINIIT12 module continuously monitors the primary over dedicated Controlway. A failover occurs when the secondary module detects a primary module failure. When this happens, the secondary interface takes over and the primary interface is taken offline.INIIL02 Local InterfaceThe INIIL02 Local Interface consists of two INNIS01 Network Interface modules and the INIIT03 Local Transfer Module (Fig.3). This interface acts as a bridge between two local Cnets. One of the INNIS01 modules operates on the central network side and the other operates on the satellite net-work side. Bidirectional communication from the central network to the local satellite network is through cable connection to the NTCL01 termination unit. The maximum distance betweentermination units on the two communication networks is 45.8 meters (150feet).The local interface supports hardware redundancy. Redundancy requires a full set of duplicatemodules (four INNIS01 modules and two INIIT03 modules). The secondary INIIT03 module con-tinuously monitors the primary over dedicated Controlway. A failover occurs when the secondary detects a primary module failure. When this happens, the secondary assumes responsibility and the primary is taken offline.Cnet-to-HCU Communication InterfaceThe Harmony control unit interface consists of the INNIS01 Network Interface Module and the INNPM12 or INNPM11 Network Processing Module (Fig. 4). This interface can be used for a node on the central network or on a satellite network (Fig.1). Through this interface the Harmony con-trol unit has access to Cnet and to Controlway at the same time. Controlway is an internal cabinet communication bus between Harmony rack controllers and the communication interfacemodules.The HCU interface supports hardware redundancy. Redundancy requires a full set of duplicate modules (two INNIS01 modules and two INNPM12 or INNPM11 modules). The secondary net-work processing module (INNPM12 or INNPM11) continuously monitors the primary through a direct ribbon cable connection. A failover occurs when the secondary detects a primary module failure. When this happens, the secondary assumes responsibility and the primary is taken offline. Cnet-to-Computer Communication InterfaceThe Cnet-to-computer interfaces are the INICI03 and INICI12 interfaces. The INICI03 interfaceconsists of the INNIS01 Network Interface Module, the INICT03A Computer Transfer Module,and the IMMPI01 Multifunction Processor Interface Module (Fig. 5). The INICI12 interface con-sists of the INNIS01 Network Interface Module and the INICT12 Computer Transfer Module(Fig6).Harmony Rack CommunicationsFigure 4. Cnet-to-HCU InterfaceFigure 5. Cnet-to-Computer Interface (INICI03)Figure 6. Cnet-to-Computer Interface (INICI12)Harmony Rack CommunicationsA computer interface can be used for a node on the central network or on a satellite network (Fig.1). It gives a host computer access to point data over Cnet. The computer connects through either an RS-232-C serial link at rates up to 19.2 kilobaud or through a SCSI parallel port when using an INICI03 interface. The computer connects through an RS-232-C serial link at rates up to 19.2 kilobaud when using an INICI12 interface. Each interface is command driven through soft-ware on the host computer. It receives a command from the host computer, executes it, then replies to the host computer.Note: A workstation running Conductor VMS software does not use an INICI03 or INICI12 Cnet-to-Computer Interface but instead has its own dedicated version of the Cnet-to-computer interface (IIMCP02 and IIMLM01).Communication ModulesTable 1 lists the available Harmony rack communication modules. These modules, in certain combinations, create the various Cnet communication interfaces.Network Interface ModuleThe INNIS01 Network Interface Module is the front end for all the different Cnet communication interfaces. It is the intelligent link between a node and Cnet. The INNIS01 module works in con-junction with the transfer modules and the network processing module. This allows any node to communicate with any other node within the Symphony system.The INNIS01 module is a single printed circuit board that occupies one slot in the module mount-ing unit (MMU). The circuit board contains microprocessor based communication circuitry that enables it to directly communicate with the transfer modules and network processing module, and to interface to Cnet.The INNIS01 module connects to its Cnet communication network through a cable connected to an NTCL01 termination unit. Communication between nodes is through coaxial or twinaxial cables that connect to the termination units on each node.Cnet-to-Cnet Remote Transfer ModuleThe INIIT12 Remote Transfer Module supports bidirectional communication through twoRS-232-C ports. Port one passes system data only. Port two passes system data or can be used as a diagnostic port. The central network INIIT12 module can use a variety of means to link to the sat-ellite network INIIT12 module such as modems, microwave, and transceivers. The INIIT12Table 1. Harmony Rack Communication Modules ModuleDescription Cnet-to-Cnet Cnet-to-HCU Cnet-to-Computer INIIR01 INIIL02 INICI03INICI12 IMMPI01Multifunction processor interface •INICT03ACnet-to-computer transfer •INICT12Cnet-to-computer transfer •INIIT03Cnet-to-Cnet local transfer •INIIT12Cnet-to-Cnet remote transfer •INNIS01Network interface •••••INNPM11 or INNPM12Network processing•Harmony Rack Communicationsmodule directly communicates with an INNIS01 module. Many of the operating characteristics of the INIIT12 module are determined by function code202 (INIIT12 executive) specifications.The INIIT12 module is a single printed circuit board that occupies one slot in the module mount-ing unit. The circuit board contains microprocessor based communication circuitry that enables it to serially communicate with another INIIT12 module, to directly communicate with its INNIS01 module, and to interface to Controlway.The INIIT12 module connects through a cable to an NTMP01 termination unit. The two RS-232-C ports are located on the termination unit.Cnet-to-Cnet Local Transfer ModuleThe INIIT03 Local Transfer Module serves as the bridge between two local Cnet communication networks. It holds the node database and is responsible for transferring all messages between net-works. Messages include exception reports, configuration data, control data, and system status.This module directly communicates with the INNIS01 module of the central network and of the satellite network simultaneously.The INIIT03 module is a single printed circuit board that occupies one slot in the module mount-ing unit. The circuit board contains microprocessor based communication circuitry that enables it to directly communicate with its two INNIS01 modules and to interface to Controlway.Cnet-to-Computer Transfer ModuleThe INICT03A Computer Transfer Module and INICT12 Computer Transfer Module handle all communication with a host computer. These modules are command driven through software on the host computer. The module receives a command from the host computer, executes it, thenreplies. Its firmware enables the host computer to issue commands for data acquisition, process monitoring, and process control, and to perform system functions such as security, timesynchronization, status monitoring, and module configuration.The INICT03A and INICT12 modules are single printed circuit boards that occupy one slot in the module mounting unit. Their capabilities and computer connection methods differ. The INICT03A module can store up to 30,000 point definitions (depending on point types). The INICT12 module can store up to 10,000 point definitions.For the INICT03A module, the circuit board contains microprocessor based communication cir-cuitry that enables it to directly communicate with its INNIS01 module and to directlycommunicate with an IMMPI01 module. It communicates with the IMMPI01 module through a ribbon cable connection. The IMMPI01 module handles the actual host computer interface andsupports RS-232-C or SCSI serial communication.For the INICT12 module, the circuit board contains microprocessor based communication cir-cuitry that enables it to directly communicate with its INNIS01 module and to directlycommunicate with a host computer using RS-232-C serial communication. The module cable con-nects to an NTMP01 termination unit. Two RS-232-C ports are located on the termination unit. The NTMP01 jumper configuration determines DTE or DCE operation.Multifunction Processor Interface ModuleThe IMMPI01 Multifunction Processor Interface Module handles the I/O interface between thehost computer and the INICT03A Computer Transfer Module. The IMMPI01 module supportseither a SCSI or RS-232-C computer interface. When communicating through the RS-232-C port, the module can act as data communication equipment (DCE) or data terminal equipment (DTE).Harmony Rack Communications The IMMPI01 module is a single printed circuit board that occupies one slot in the module mount-ing unit. The circuit board contains microprocessor based communication circuitry that enables it to communicate with its INICT03A module through a ribbon cable connection.For RS-232-C computer interface, the module cable connects to an NTMP01 termination unit. Two RS-232-C ports are located on the termination unit. The NTMP01 jumper configuration determines DTE or DCE operation. The SCSI port is located at the module faceplate. In this case, notermination unit is required.Network Processing ModuleThe INNPM12 or INNPM11 Network Processing Module acts as a gateway between Cnet andControlway. The module holds the Harmony control unit database and handles the communica-tion between controllers residing on Controlway and the INNIS01 module.The INNPM12 or INNPM11 module is a single printed circuit board that occupies one slot in the module mounting unit. The circuit board contains microprocessor based communication circuitry that enables it to directly communicate with its INNIS01 module and to interface to Controlway.Rack Communications PowerHarmony rack communication modules are powered by 5, 15, and -15VDC logic power. Modular Power System II supplies the logic power. These operating voltages are distributed from thepower system through a system power bus bar mounted in the cabinet. A module mounting unit connects to this bus bar then routes the power to individual modules through backplaneconnectors.Rack Communications Mounting HardwareHarmony rack communication modules and their termination units mount in standard ABB cabi-nets. The option for small cabinet mounting is provided. The number of modules that can bemounted in a single cabinet varies. Modules of an interface are always mounted in adjacent slots.An IEMMU11, IEMMU12, IEMMU21, or IEMMU22 Module Mounting Unit and an NFTP01 Field Termination Panel are used for module and termination unit mounting respectively (Fig. 7). The mounting unit and termination panel both attach to standard 483-millimeter (19-inch) width side rails. Front mount and rear mount MMU versions are available to provide flexibility in cabinetmounting.A module mounting unit is required to mount and provide power to rack mounted modules. Theunit is for mounting Harmony rack controllers, I/O modules, and communication interfacemodules. The MMU backplane connects and routes:■Controlway.■I/O expander bus.■Logic power to rack modules.The Controlway and I/O expander bus are internal cabinet, communication buses. Communica-tion between rack controllers and HCU communication interface modules is over Controlway. The Cnet-to-Cnet interfaces use dedicated Controlway for redundancy communication. This dedicated Controlway is isolated from all other modules.Harmony Rack CommunicationsFigure 7. Rack I/O Mounting HardwareRelated DocumentsNumber Document TitleWBPEEUD250001??Harmony Rack Communications, Data SheetHarmony Rack Communications WBPEEUS250002C111Harmony Rack CommunicationsWBPEEUS250002C1Litho in U.S.A.May 2003Copyright © 2003 by ABB, All Rights Reserved® Registered Trademark of ABB.™ Trademark of ABB.For more information on the Control IT suiteofproducts,***************************.comFor the latest information on ABB visit us on the World Wide Web at /controlAutomation Technology Products Mannheim, Germany www.abb.de/processautomation email:*********************************.com Automation Technology ProductsWickliffe, Ohio, USA/processautomation email:****************************.com Automation Technology Products Västerås, Sweden /processautomation email:************************.com ™Composer, Control IT , and Symphony are trademarks of ABB.。
英语作文-集成电路设计的关键成功因素与市场竞争策略
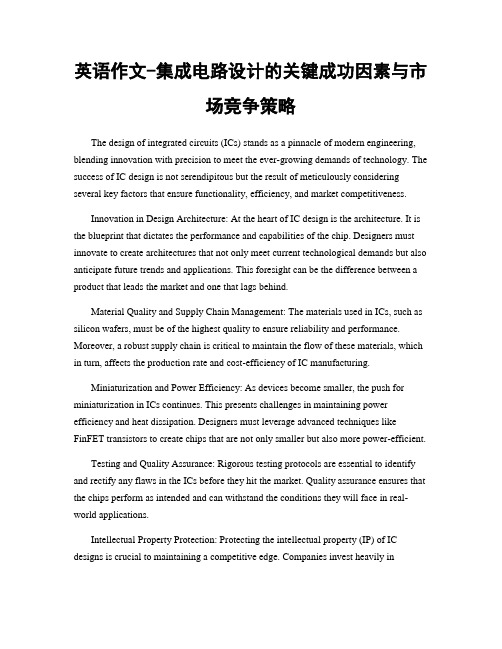
英语作文-集成电路设计的关键成功因素与市场竞争策略The design of integrated circuits (ICs) stands as a pinnacle of modern engineering, blending innovation with precision to meet the ever-growing demands of technology. The success of IC design is not serendipitous but the result of meticulously considering several key factors that ensure functionality, efficiency, and market competitiveness.Innovation in Design Architecture: At the heart of IC design is the architecture. It is the blueprint that dictates the performance and capabilities of the chip. Designers must innovate to create architectures that not only meet current technological demands but also anticipate future trends and applications. This foresight can be the difference between a product that leads the market and one that lags behind.Material Quality and Supply Chain Management: The materials used in ICs, such as silicon wafers, must be of the highest quality to ensure reliability and performance. Moreover, a robust supply chain is critical to maintain the flow of these materials, which in turn, affects the production rate and cost-efficiency of IC manufacturing.Miniaturization and Power Efficiency: As devices become smaller, the push for miniaturization in ICs continues. This presents challenges in maintaining power efficiency and heat dissipation. Designers must leverage advanced techniques like FinFET transistors to create chips that are not only smaller but also more power-efficient.Testing and Quality Assurance: Rigorous testing protocols are essential to identify and rectify any flaws in the ICs before they hit the market. Quality assurance ensures that the chips perform as intended and can withstand the conditions they will face in real-world applications.Intellectual Property Protection: Protecting the intellectual property (IP) of IC designs is crucial to maintaining a competitive edge. Companies invest heavily inresearch and development, and safeguarding these investments through patents and trade secrets is vital for sustaining innovation and market position.Market Analysis and Consumer Needs: Understanding the market is key to successful IC design. This involves analyzing consumer needs, staying ahead of technological trends, and predicting future demands. A design that aligns with market needs will have a better chance of success.Cost Optimization: While innovation is important, it must be balanced with cost. The IC design process should aim to optimize costs without compromising on quality. This includes considering manufacturing processes that can scale efficiently and reduce waste.Collaboration and Teamwork: The complexity of IC design often requires a multidisciplinary approach. Collaboration among experts in different fields, such as electrical engineering, material science, and computer science, is essential to create a product that is innovative, efficient, and market-ready.Adaptability to Changing Technologies: The tech industry is dynamic, with rapid changes in technologies and consumer preferences. IC designs must be adaptable, allowing for updates and modifications to extend the product's lifecycle and relevance in the market.Regulatory Compliance: Compliance with international standards and regulations is non-negotiable. IC designs must adhere to these standards to ensure safety, compatibility, and the ability to be marketed globally.In conclusion, the key to successful IC design lies in a harmonious blend of innovation, quality, market insight, and adaptability. By focusing on these factors, designers can create ICs that not only perform exceptionally but also stand strong in the competitive market. The strategies employed must be dynamic, reflecting the ever-evolving landscape of technology and consumer expectations. It is through this meticulous approach that the ICs of tomorrow will power the future of technology. 。
有关芯片英语作文

有关芯片英语作文The chip is like the brain of a computer, it controls everything and makes everything work. Without the chip, the computer would be like a body without a brain, it wouldn't be able to do anything.Chips are used in so many things, not just computers. They are in our phones, our cars, our TVs, and even in some of our kitchen appliances. They make all of these things work and make our lives easier.The technology in chips is always improving. New chips are faster, smaller, and more powerful than ever before. This means that the devices we use can do more things and do them faster. It's amazing to think about how much power is packed into such a small space.Chips are made using a process called "semiconductor manufacturing." This is a very complex process that involves creating tiny circuits on a piece of silicon. It'sincredible to think about how something so small can have such a big impact on our lives.In the future, chips will continue to get even smaller and more powerful. This means that the devices we use will continue to improve and become even more integrated into our daily lives. It's exciting to think about what the future holds for chip technology.。
表达谱芯片array
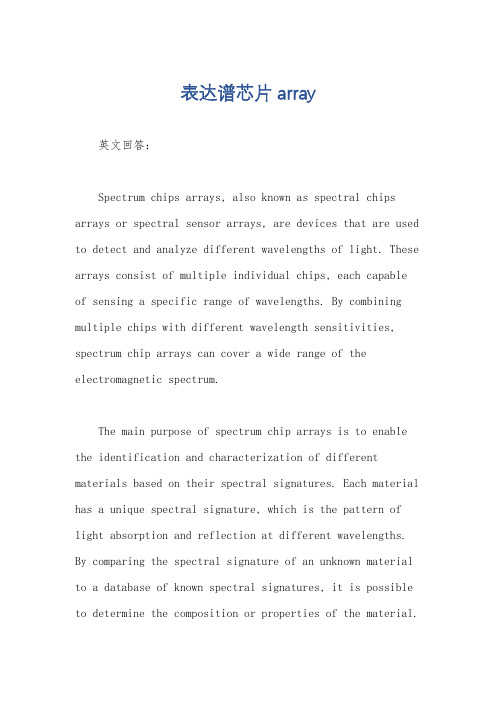
表达谱芯片array英文回答:Spectrum chips arrays, also known as spectral chips arrays or spectral sensor arrays, are devices that are used to detect and analyze different wavelengths of light. These arrays consist of multiple individual chips, each capable of sensing a specific range of wavelengths. By combining multiple chips with different wavelength sensitivities, spectrum chip arrays can cover a wide range of the electromagnetic spectrum.The main purpose of spectrum chip arrays is to enable the identification and characterization of different materials based on their spectral signatures. Each material has a unique spectral signature, which is the pattern of light absorption and reflection at different wavelengths. By comparing the spectral signature of an unknown material to a database of known spectral signatures, it is possible to determine the composition or properties of the material.One common application of spectrum chip arrays is in environmental monitoring. For example, these arrays can be used to analyze the composition of water samples, allowing scientists to detect pollutants or contaminants. Another application is in agriculture, where spectrum chip arrays can be used to monitor the health and nutrient content of crops.In addition to their scientific and industrial applications, spectrum chip arrays also have potential consumer applications. For instance, these arrays can be used in smartphones to enable advanced camera features, such as accurate color reproduction and automatic white balance adjustment. They can also be used in wearable devices for fitness tracking, by analyzing the user's blood oxygen levels through the detection of specific wavelengths of light.Overall, spectrum chip arrays are versatile devicesthat have a wide range of applications. They enable the detection and analysis of different wavelengths of light,allowing for the identification and characterization of materials. Whether in scientific research, industrial processes, or consumer electronics, spectrum chip arraysplay a crucial role in understanding and utilizing the electromagnetic spectrum.中文回答:谱芯片阵列,也称为光谱芯片阵列或光谱传感器阵列,是用于检测和分析不同波长的光的设备。
有关集成电路的英语作文
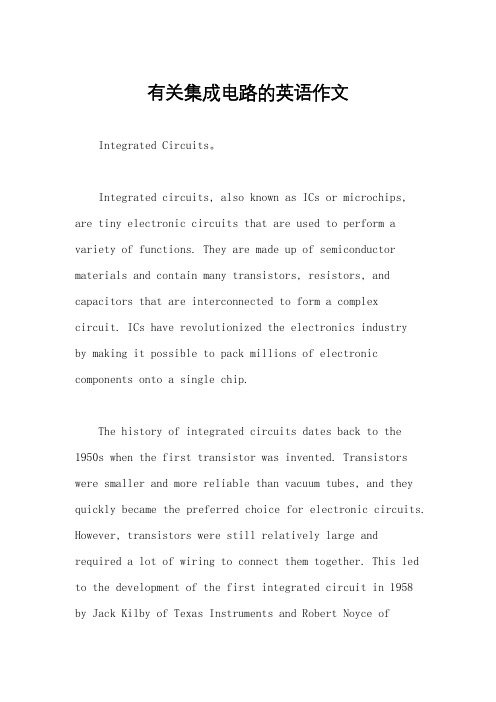
有关集成电路的英语作文Integrated Circuits。
Integrated circuits, also known as ICs or microchips, are tiny electronic circuits that are used to perform a variety of functions. They are made up of semiconductor materials and contain many transistors, resistors, and capacitors that are interconnected to form a complexcircuit. ICs have revolutionized the electronics industryby making it possible to pack millions of electronic components onto a single chip.The history of integrated circuits dates back to the 1950s when the first transistor was invented. Transistors were smaller and more reliable than vacuum tubes, and they quickly became the preferred choice for electronic circuits. However, transistors were still relatively large and required a lot of wiring to connect them together. This led to the development of the first integrated circuit in 1958 by Jack Kilby of Texas Instruments and Robert Noyce ofFairchild Semiconductor.The first integrated circuit was a simple device that contained only a few transistors, resistors, and capacitors. However, it paved the way for the development of more complex ICs that could perform a variety of functions. Bythe 1960s, ICs were being used in a wide range of applications, including computers, calculators, and telecommunications equipment.Today, ICs are used in almost every electronic device, from smartphones and laptops to cars and airplanes. Theyare used to control everything from the temperature of your coffee maker to the speed of your car. ICs have also madeit possible to create tiny sensors that can detect everything from light and sound to temperature and pressure.There are many different types of ICs, each designed to perform a specific function. Some of the most common typesof ICs include microprocessors, memory chips, and analog circuits. Microprocessors are used in computers and other digital devices to control the flow of data and performcalculations. Memory chips are used to store data and program instructions, while analog circuits are used to process signals such as sound and video.In conclusion, integrated circuits have had a profound impact on the electronics industry and have made it possible to create smaller, faster, and more powerful electronic devices. They have revolutionized the way we live and work, and they will continue to play a critical role in shaping the future of technology.。
- 1、下载文档前请自行甄别文档内容的完整性,平台不提供额外的编辑、内容补充、找答案等附加服务。
- 2、"仅部分预览"的文档,不可在线预览部分如存在完整性等问题,可反馈申请退款(可完整预览的文档不适用该条件!)。
- 3、如文档侵犯您的权益,请联系客服反馈,我们会尽快为您处理(人工客服工作时间:9:00-18:30)。
Networks on Chips:A Synthesis Perspective F.Angiolini,P.Meloni,D.Bertozzi,L.Benini,S.Carta,L.Raffopublished inParallel Computing:Current&Future Issues of High-End Computing,Proceedings of the International Conference ParCo2005,G.R.Joubert,W.E.Nagel,F.J.Peters,O.Plata,P.Tirado,E.Zapata (Editors),John von Neumann Institute for Computing,J¨ulich,NIC Series,Vol.33,ISBN3-00-017352-8,pp.745-752,2006.c 2006by John von Neumann Institute for ComputingPermission to make digital or hard copies of portions of this work for personal or classroom use is granted provided that the copies are not made or distributed for profit or commercial advantage and that copies bear this notice and the full citation on thefirst page.To copy otherwise requires prior specific permission by the publisher mentioned above.http://www.fz-juelich.de/nic-series/volume33745Networks on Chips:A Synthesis PerspectiveFederico Angiolini a,Paolo Meloni b,Davide Bertozzi c,Luca Benini a,Salvatore Carta d,LuigiRaffo ba Dipartimento di Elettronica,Informatica e Sistemistica,University of Bologna,40136Bologna, Italyb Dipartimento di Ingegneria Elettrica ed Elettronica,University of Cagliari,09123Cagliari,Italyc Dipartimento di Ingegneria,University of Ferrara,44100Ferrara,Italyd Dipartimento di Matematicae Informatica,University of Cagliari,09123Cagliari,ItalyTo face increasing requirements for computational density in embedded chips,MultiProcessor Systems-on-Chip(MPSoCs)are being widely deployed.This evolution increases communication re-quirements,therefore new,more scalable,on-chip interconnect fabrics are being called works-on-Chip(NoCs)appear to solve the upcoming scalability issue.However,it is presently unclear how exactly NoCs can position themselves in terms of performance/area tradeoff.Since NoCs are supposed to span across the whole chip area,difficult questions associated to chip layout arise.Specifically,the delay impact of long-range wiring resources is unknown,and the delay estimation provided by synthesis tools must be verified against post-placementfigures.This paper will address such question marks,by showing a complete NoC synthesisflow going down to the layout level.The experimental results include assessment of a complete placed NoC instance and analysis of single switches when synthesized in varying configurations.1.IntroductionThe ever increasing System-on-Chip(SoC)integration is calling for a huge increase in intra-chip communication resources.The traditional SoC interconnect fabric leverages upon a single shared bus;this approach is quickly becoming insufficient to cope with current and future requirements. More complex and better performing architectures,namely crossbars,are being studied and de-ployed,but they are inherently non-scalable due to wiring congestion.This fact dictates the need for a more radical departure from the common notion of a monolithic communication fabric featuring afixed topology.Instead,packet-switched networks,such as the Internet,spring to mind for their efficiency and inherent scalability.The transfer of the packet-switching concept to the on-die realm takes the name of Network-on-Chip(NoC).NoCs provide aflexible path toward complex fabric topologies with abundant amounts of available bandwidth.SoC architectures exhibit a highly heterogeneous nature,and industry trends point at the integra-tion of an increasing number of different functional blocks on the same die.Therefore,homogeneous or preconfigured NoCs look like an inefficient solution to the interconnect scalability problem.This observation calls for the creation of a library of highly parameterizable NoC components,enabling the designer to instantiate a NoC architecture custom-tailored to specific applications and usage needs.The configurability of such“soft macros”however must be provided without incurring in any noticeable performance overhead.While undertaking the effort of developing such building blocks,it is of course crucial to assess the performance,area and power targets of the whole NoC instance.This task is not trivial,also746due to the unknown wiring overhead after performing thefinal chip placement and routing.Such overhead is clearly critical in a distributed component spanning the whole chip area.Thus,a key design principle must be a strict control of wiring resources,so that they do not span across long distances on the die.This can be achieved e.g.by link pipelining through clocked repeaters.In any case,it is essential to be able to carry the design through all stages of physical synthesis before being able to properly estimate critical factors such as maximum achievable clock frequency.The presently available industrial and academic literature is lacking thorough examples of this analysis.This work will discuss the above key topic by presenting synthesis results for×pipes,a library of fully synthesizable,highly customizable,high frequency and low latency NoC modules.By leverag-ing upon aflexible custom-built CAD toolchain,we enabled a completeflow where the×pipes com-ponents were deployed in a NoC topology interconnecting functional IP cores.This topology,which is fully simulatable to assess architectural efficiency,was then synthesized in a0.13µm technology, andfinally placed and routed.The last step allowed us to get reliablefigures about critical parame-ters such as frequency and area of the NoC.In this work,we will mostly focus on the description of a complete topology instantiation and on the area/delay issues of wiring resources.A thorough power consumption analysis,while possible in the framework that we will describe,requires additional research to identify appropriate traffic patterns for the modeling of an entire topology and of single building blocks,and is therefore left for future work.This paper is organized as follows.Section2will discuss the state of the art in NoC implemen-tations.Section3will describe the component blocks of the NoC we developed and synthesized. Section4will describe the synthesis steps and related issues,while Section5will show experimental results.Finally,Section6will draw conclusions.2.Related WorkTo face the interconnect scalability bottleneck,NoCs have been suggested as an effective long-term solution[8],[3].In recent years,an increasingly large body of research has bloomed around this subject,focusing for example on complete architectures[11],flow control protocols[13],Quality of Service(QoS)provisions[6],[16],support CAD tools[4],asynchronous implementations[5]. While these issues are critical,they are often discussed without a complete analysis of the area and delay implications when carrying such designs onto a real chip.Work focused on the synthesis stage of the NoCflow exists.For example,a test chip,mostly focused on electrical properties and power consumption,is mentioned in[12],while fully placed designs are presented in[14],[2]. However,neither of them proposes a completely configurable library of parameterizable building blocks,neither presents wide spectrum architectural exploration results,and all rely on some full custom blocks to achieve higher performance.For some NoCs[17],FPGA mappings are described in the literature.However,these attempts help shedding light only up to a certain extent,due to the huge unpredictability of the FPGA synthesis results across different device families and vendors. Moreover,physical delay issues get masked by the architecture of the specific FPGA chip at hand.We build on our previous work[7],[15]to further illustrate a fully parametric NoC library,car-rying its implementation to the layout level.While still being able to explore architectural design tradeoffs thanks to the completely synthetic nature of theflow,this step makes it possible to evaluate important design assumptions,such as wire predictability and scalability.747 3.×pipes Architectural Blocks×pipes is a highly configurable,high performance NoC.One of its most prominent features is the ability to be deployed in different topologies and with different design parameters,thus matching the requirements of any specific target application.To achieve this objective,×pipes is based upon a library of components;it is the system designer’s responsibility to pick up,configure and connect the proper blocks to create the complete interconnect fabric,of course assisted by CAD tools.The ×pipes library contains three main components:switches,Network Interfaces(NIs)and links. Switches constitute the backbone of the NoC,as they route packets from sources to destinations. They can be connected in aflexible manner,according to the designer’s preference,thus resulting in arbitrary fabric topologies.Switches provide buffering resources to improve performance;in×pipes, output buffering was chosen,i.e.FIFOs are present on each output port.Moreover,switches handle flow control issues,and resolve conflicts among packets when they overlap in requesting access to the same physical links.NIs take care of the task of converting processor transaction requests into packets,and of the dual process of transaction unpacketing.Therefore,an NI is needed to connect each IP core to the NoC.Further,each packet is split by the NIs into a sequence of“flits”(FLow control unITS) before transmission,to decrease the physical wire parallelism requirements.In×pipes,two separate NIs are defined,an initiator and a target one,respectively associated to system masters and system slaves.In case of a device requiring both interfaces,an NI of each type must be attached to it.The interface among IP cores and NIs is defined by the OCP2.0[1]specification,for maximum reuse capability.NIs also take care of specifying the path that packets will follow in the network to reach their destination(source routing),by means of path Look-Up Tables(LUTs).NIs provide dual clock capability,in that two different clock signals can be attached to it:one is driving the NI front-end(the OCP interface),the other is driving the NI back-end(the×pipes interface).These clocks have to be in an integer multiple relationship with one another,the OCP clock being slower.This arrangement allows the NoC fabric to run at a fast clock even though some or all of the attached IP cores are slower,which is crucial to keep transaction latency low.Also,each IP core can run at a different divider of the×pipes frequency,therefore making mixed-clock platforms possible.Inter-block links are a critical component of NoCs,especially when taking into account technol-ogy trends.Since links can span across the whole die or significant portions thereof,the problem of signal propagation delay is critical.For this reason,×pipes supports link pipelining,i.e.the interleaving of logical buffers along links.Properflow control protocols are implemented in link transmitters and receivers,i.e.within NIs and switches,to make the link latency transparent to the surrounding logic.By means of this design choice,the overall platform can run at a fast clock fre-quency,without the longest wires being a global speed limiter.Instead,only the links which are too long for single-cycle propagation will pay a latency penalty.A custom CAD tool,called×pipesCompiler,allows the designer to instantiate and configure ×pipes library components tofit his or her application needs.By means of a simple specification textfile(which may be generated as the output of an additional external tool),components can be attached to each other in arbitrary topologies,and routing tables can be freely set.The NoCflow control policy can be chosen among a simple single-token credit-based one(called STALL/GO),and a retransmission-enabled one(called ACK/NACK),which provides some of the facilities required for more thorough fault tolerance.Theflit width can be set to arbitrary values.Additionally,switches can be customized in terms of I/O ports,buffering resources and arbitration policies,while network interfaces in terms of buffering resources and clock dividers.7484.Toward Chip LayoutOne of the aims of the×pipes NoC infrastructure is devising a complete designflow,letting the designer configure,simulate,synthesize and verify an MPSoC instance.For this paper,we willstages.especially focus on the portions of thisflow which pertain to the synthesis and place&route Array Figure1.The×pipes designflowFigure1is showing a high-level view of the complete process of instantiating a NoC platform. The main inputs of theflow are the×pipes component library,written in SystemC,and a text for-mat topology specification.The×pipesCompiler tool processes these sources to generate a SystemC instance,which is suitable both for architectural simulation and for synthesis.When the synthesis path is taken,a SystemC-to-HDL automatic translation is performed.Three major outputs are the result:the HDL code for traffic generators,for the NoC building blocks,and for the top level in-stantiation code.The latter two are synthesized with Synopsys Design Compiler[10].During this stage,a technology library is required;for this paper,we used a0.13µm library.No full custom blocks are employed in the process,even though they would lead to better performance,to illustrate the results achievable with a completely synthetic,and therefore maximallyflexible,approach.The resulting netlists now need to be placed and routed,which is done in two steps by Cadence SoC Encounter[9].First,each building block is processed;subsequently,blocks are connected together according to the top level module specifications,and thefinal optimizations are made.Thefinal output is a placed&routed topology,which is suitable for further area and delay analysis and for ver-ification.By back annotation of parasitic capacitances,powerfigures could be extracted,but such analysis is beyond the scope of this paper.5.Experimental ResultsFigure2(b)depicts a sample NoCfloorplan resulting from the previously describedflow.For this paper,we chose a5x3regular mesh(Figure2(a)),which interconnects30IP cores(15masters and749(a)(b)Figure2.Topology(a)andfloorplan(b)of a5x3×pipes mesh15slaves).Each of these cores is supposed to be square and obstructing an area of1mm2,which is reasonable for embedded cores and/or32kB SRAM banks at the0.13µm node.Synthesis was car-ried on by leveraging a UMC0.13µm technology library,which does not have extreme performance as its focus,but for which obstruction information was available to us in the place&route phase.Our test topology comprises30NIs,equally split among initiators and targets,and15switches,of which three having6input and6output ports(those in the center of the mesh),eight5x5(at the sides),and four4x4(in the corners).Each switch is connected to one initiator and one target NI.The mesh is configured with38-bitflits,3-flit buffering FIFOs,fixed priority arbitration,the ACK/NACKflow control protocol,and non-pipelined links.Experimental results for this topology are reported in Fig-ure3at varying frequency targets.As can be seen,even when pushed to the performance limits,the NoC cells do not require more than7%of the overall die area.At a lower frequency target of500 MHz,NoC cells take5.7%of the chip real estate.However,wiring resources and the need to reserve some slack space for the global nets(e.g.the clock tree)cause the baseline area of30mm2(30IP cores of1mm2each)to stretch to a global area of42to44mm2.We would like to mention that wework.did not perform any specific area optimization in this ArrayFigure3.Area of a5x3×pipes mesh synthesized with different frequency targetsAn importantfigure to notice here is the degree of divergence among delay estimations based upon the netlist and upon the placed topology.When aiming for maximum frequency,the netlist was estimated to be capable of running at910MHz;after the place&route stage,the real achievable frequency was845MHz,which translates into a decrease of just8%.Moreover,the critical path was within the NoC logic(namely,the6x6switches),and not on the global wires,which even allowed us to deploy non-pipelined links among the NoC blocks.Thesefindings confirm the effectiveness of NoCs in downplaying global wires as performance limiters.Figure4analyzes the behaviour of switch components when synthesized with varying configura-750tion parameters.We will explore the configuration space independently for each parameter,keeping as a baseline a6x6,38-bit switch having a3-flit buffer depth and usingfixed priority arbitration and ACK/NACKflow control.All instances have been synthesized,placed and eventually routed to providefigures as accurate as possible.Figures4(a)and4(b)show trends when varying the amount of I/O ports;since the bulk of the switch logic and buffering in×pipes is associated with output ports,which is compounded by a mild dependence on inputs,the area scales up a bit more than linearly with the amount of output ports. Increasing numbers of input ports make arbitration and multiplexing more complex,which results in a10%worse frequency when moving from four to six inputs.Figures4(c)and4(d)depict performance when varying theflit width of packets.When moving from16to38bits(which is an optimalflit size for performance once the decomposition of packets intoflits is taken into account),a huge area penalty of64%can be observed.Such penalty is however weakly proportional toflit width,which increased by138%.This result is logical,since the area for the datapath(including buffers)has to scale linearly withflit width,but arbitration and control logic are unaffected.The maximum operating frequency is also almost unaffected byflit width,which suggests the worsening of wiring congestion to be not critical.The impact of buffering is investigated in Figures4(e)and4(f).Since buffering resources repre-sent a significant percentage of the component area,doubling the buffer depth results in a noticeable 54%area penalty.Also,due to the FIFO nature of the buffers,increased logic and wiring complexity impacts maximum frequency by as much as52MHz(around6%).Implementing a fair round-robin arbitration policy instead of the baselinefixed priority one incurs a noticeable cost.Additional logic to track the status of input ports results in15%worse area and maximum operating frequency(Figures4(g)and4(h)).The choice offlow control protocols impacts the performance of the NoC also with respect to performance of the single building blocks.We tested with two alternative protocols,namely ACK/NACK(which features retransmission capabilities,and is suitable for fault-tolerant environ-ments)and STALL/GO(which is a variant of credit-based schemes where only one credit is avail-able,thus allowing for a pipelined link implementation).The×pipes architecture natively exhibits output buffering,and is therefore more suitable to the ACK/NACK protocol.When deploying STALL/GO logic,additional input buffering had to be added;albeit minimal,this incurred a52% area overhead and a5%lower frequency(Figures4(i)and4(j)).6.Conclusions and Future WorkIn this paper,we showed a complete NoC synthesisflow leading to chip layout.Synthesis results highlight the relationship among design parameters andfinal performance,helping the designer to choose the best tradeoffs.As an example,for the×pipes architecture,to achieve maximum perfor-mance if area is not a concern,increasing theflit widthfirst is better than adding buffers,since it has a much lower frequency penalty.The place&route phase is essential to validate the proposedflow and to check design assumptions. Despite of the distributed nature of NoC fabrics,post-place&route synthesisfigures do not show a significant delay degradation with respect to netlist estimates,and critical paths are within the building blocks.When considering that traditional interconnects are limited by global wire delay already at the current lithographic nodes,these twofindings strengthen the positioning of NoCs as a highly scalable,highly predictable interconnect fabric for future technologies.Future work revolves around architectural optimizations,a thorough power consumption analysis and the investigation of the performance impact of the usage of full custom logic blocks.751 References[1]Open Core Protocol Specification,Release2.0,,2003.[2]Adrijean Andriahantenaina and Alain Greiner.Micro-network for SoC:Implementation of a32-portspin network.In The Proceedings of Design,Automation and Test in Europe Conference and Exhibition, pages1128–1129.IEEE,2003.[3]Luca Benini and Giovanni De works on chips:A new SoC paradigm.IEEE Computer,35(1):70–78,January2002.[4]Davide Bertozzi,Antoine Jalabert,Srinivasan Murali,Rutuparna R.Tamhankar,Stergios Stergiou,LucaBenini,and Giovanni De Micheli.NoC synthesisflow for customized domain specific multiprocessor systems-on-chip.IEEE Transactions on Parallel and Distributed Systems,16,Issue2:113–129,Febru-ary2005.[5]Tobias Bjerregaard and Jens Sparsø.Scheduling discipline for latency and bandwidth guarantees in asyn-chronous network-on-chip.In Proceedings of the11th IEEE International Symposium on Asynchronous Circuits and Systems(ASYNC),pages34–43,2005.[6]Evgeny Bolotin,Israel Cidon,Ran Ginosar,and Avinoam Kolodny.QNoC:QoS architecture and designprocess for network on chip.In Journal of Systems Architecture.Elsevier,2004.[7]Matteo Dall’Osso,Gianluca Biccari,Luca Giovannini,Davide Bertozzi,and Luca Benini.×pipes:Alatency insensitive parameterized Network-on-Chip architecture for multi-processor SoCs.In Proceed-ings of21st International Conference on Computer Design,pages536–539.IEEE Computer Society, 2003.[8]William J.Dally and Brian Towles.Route packets,not wires:On-chip interconnection networks.InProceedings of the38th Design Automation Conference,pages684–689,June2001.[9]Cadence Design Systems Inc.SoC Encounter,.[10]Synopsys Inc.Design Compiler,.[11]Faraydon Karim,Anh Nguyen,Sujit Dey,and Ramesh Rao.On-chip communication architecture forOC-768network processors.In Proceedings of the Design Automation Conference(DAC),pages678–683,2001.[12]Kangmin Lee,Se-Joong Lee,Sung-Eun Kim,Hye-Mi Choi,Donghyun Kim,Sunyoung Kim,Min-WukLee,and Hoi-Jun Yoo.A51mW1.6GHz on-chip network for low-power heterogeneous SoC platform.In Digest of Technical Papers of the2004IEEE International Solid-State Circuits Conference(ISSC), pages152–518.IEEE Computer Society,2004.[13]Antonio Pullini,Federico Angiolini,Davide Bertozzi,and Luca Benini.Fault tolerance overhead innetwork-on-chipflow control schemes.In Proceedings of the SBCCI Conference2005(to be published), 2005.[14]Andrei Radulescu,John Dielissen,Kees Goossens,Edwin Rijpkema,and Paul Wielage.An efficienton-chip network interface offering guaranteed services,shared-memory abstraction,andflexible net-work configuration.In Proceedings of the2004Design,Automation and Test in Europe Conference (DATE’04).IEEE,2004.[15]Stergios Stergiou,Federico Angiolini,Salvatore Carta,Luigi Raffo,Davide Bertozzi,and Giovanni DeMicheli.×pipes Lite:A synthesis oriented design library for networks on chips.In Proceedings of Design,Automation and Testing in Europe Conference2005(DATE05),pages1188–1193.IEEE,March 2005.[16]Daniel Wiklund and Dake Liu.SoCBUS:Switched network on chip for hard real time embedded sys-tems.In Proceedings of the International Parallel and Distributed Processing Symposium(IPDPS03).IEEE,2003.[17]Cesar Albenes Zeferino and Altamiro Amadeu Susin.SoCIN:A parametric and scalable network-on-chip.In Proceedings of the16th Symposium on Integrated Circuits and Systems Design(SBCCI03), pages34–43,2003.(a)(b)(c)(d)(e)(f)(g)(h)(i)(j)Figure4.Area and max frequency of×pipes switches configured with varying parameters:number of ports(a-b),flit width(c-d),buffering(e-f),arbitration policy(g-h),flow control protocol(i-j) 752。