Abstract — The Reliable Server Pooling (RSerPool) protocol
《强化学习理论与应用》基于AC框架的深度强化学习方法
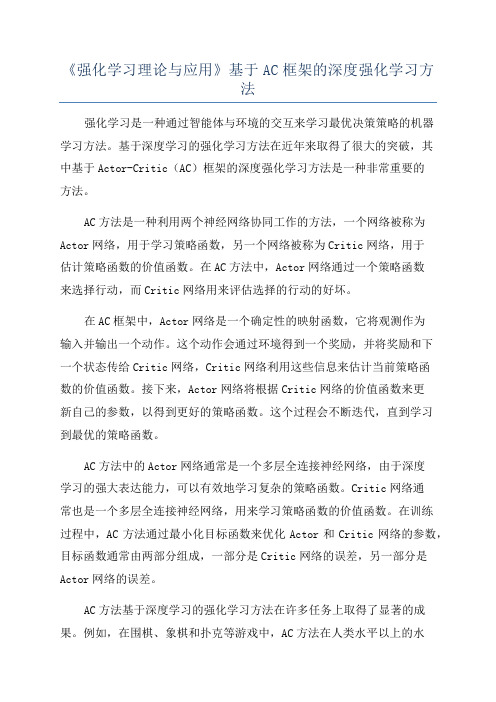
《强化学习理论与应用》基于AC框架的深度强化学习方法强化学习是一种通过智能体与环境的交互来学习最优决策策略的机器学习方法。
基于深度学习的强化学习方法在近年来取得了很大的突破,其中基于Actor-Critic(AC)框架的深度强化学习方法是一种非常重要的方法。
AC方法是一种利用两个神经网络协同工作的方法,一个网络被称为Actor网络,用于学习策略函数,另一个网络被称为Critic网络,用于估计策略函数的价值函数。
在AC方法中,Actor网络通过一个策略函数来选择行动,而Critic网络用来评估选择的行动的好坏。
在AC框架中,Actor网络是一个确定性的映射函数,它将观测作为输入并输出一个动作。
这个动作会通过环境得到一个奖励,并将奖励和下一个状态传给Critic网络,Critic网络利用这些信息来估计当前策略函数的价值函数。
接下来,Actor网络将根据Critic网络的价值函数来更新自己的参数,以得到更好的策略函数。
这个过程会不断迭代,直到学习到最优的策略函数。
AC方法中的Actor网络通常是一个多层全连接神经网络,由于深度学习的强大表达能力,可以有效地学习复杂的策略函数。
Critic网络通常也是一个多层全连接神经网络,用来学习策略函数的价值函数。
在训练过程中,AC方法通过最小化目标函数来优化Actor和Critic网络的参数,目标函数通常由两部分组成,一部分是Critic网络的误差,另一部分是Actor网络的误差。
AC方法基于深度学习的强化学习方法在许多任务上取得了显著的成果。
例如,在围棋、象棋和扑克等游戏中,AC方法在人类水平以上的水平上获得了很大的成功。
此外,AC方法还在机器人控制、交通控制和金融投资等领域中取得了重要的应用。
但是,AC方法也存在一些挑战和限制。
首先,AC方法在训练过程中需要大量的交互数据,在一些任务上可能需要很长时间才能得到满意的结果。
其次,AC方法很容易受到训练数据的质量和分布的影响,当训练数据不足或者分布不平衡时,AC方法的性能会受到限制。
无尺度半分布式P2P僵尸网络的构建

(. ol eo C mp trScu n r l ies yChn d 10 8 C ia 1C l g f o ue, i a ma v ri, eg u6 0 6 , h ; e h No Un t n
2 Istto Co uigT cn lg, h ee ae f cec sBe ig10 8 , hn ) .ntue f mp t eh oo yC i s Acd myo S i e, i 0 0 0 C ia i n n n j n
好 的 mo 台主机设置为 sret os evn t,选择时根据主机 的 I b P地 址情况、 防火墙强弱、带宽等进行筛选 。
中曩 分类号t P9 33 T
羌 尺度 半 分布 式 P P僵 尸 网络 的构 建 2
黄 彪 ,谭 良
(.四川师范大 学计算机学 院,成都 6 0 6 ;2 1 10 8 .中国科学 院计算技术研究所 ,北京 10 8 ) 000 摘 要: 鉴于僵尸 网络具有 无尺 度网络 的增长性 和择 优连接性 ,提出一种 无尺度半分布 式 P P 2 僵尸 网络 的构建 方法 。 僵尸程序在 初始时只
第 3 卷 第 1 期 8 1
、0 . 8 , 13
・
计
算
机
工
程
21 0 2年 6月
Jn 0 2 u e2 1
N o. 1 1
Co u e n i e r g mp trE g n e i n
安 全技 术 ・
文章编号tl0_ 4802 l_1 _ 3 文献标识码l 0m 32( 1l 03 _ 2 )— m 0 A
分析 ,可 以得 出模型 的度分布为 :
2 2 m t
=
人工智能基础(习题卷9)
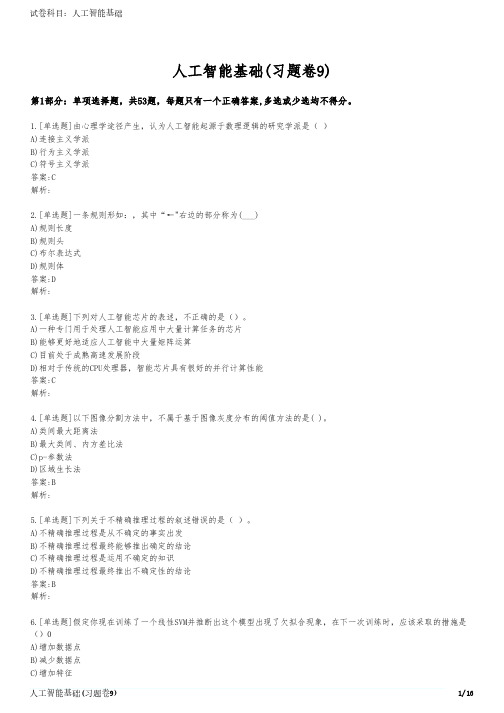
人工智能基础(习题卷9)第1部分:单项选择题,共53题,每题只有一个正确答案,多选或少选均不得分。
1.[单选题]由心理学途径产生,认为人工智能起源于数理逻辑的研究学派是( )A)连接主义学派B)行为主义学派C)符号主义学派答案:C解析:2.[单选题]一条规则形如:,其中“←"右边的部分称为(___)A)规则长度B)规则头C)布尔表达式D)规则体答案:D解析:3.[单选题]下列对人工智能芯片的表述,不正确的是()。
A)一种专门用于处理人工智能应用中大量计算任务的芯片B)能够更好地适应人工智能中大量矩阵运算C)目前处于成熟高速发展阶段D)相对于传统的CPU处理器,智能芯片具有很好的并行计算性能答案:C解析:4.[单选题]以下图像分割方法中,不属于基于图像灰度分布的阈值方法的是( )。
A)类间最大距离法B)最大类间、内方差比法C)p-参数法D)区域生长法答案:B解析:5.[单选题]下列关于不精确推理过程的叙述错误的是( )。
A)不精确推理过程是从不确定的事实出发B)不精确推理过程最终能够推出确定的结论C)不精确推理过程是运用不确定的知识D)不精确推理过程最终推出不确定性的结论答案:B解析:6.[单选题]假定你现在训练了一个线性SVM并推断出这个模型出现了欠拟合现象,在下一次训练时,应该采取的措施是()0A)增加数据点D)减少特征答案:C解析:欠拟合是指模型拟合程度不高,数据距离拟合曲线较远,或指模型没有很好地捕 捉到数据特征,不能够很好地拟合数据。
可通过增加特征解决。
7.[单选题]以下哪一个概念是用来计算复合函数的导数?A)微积分中的链式结构B)硬双曲正切函数C)softplus函数D)劲向基函数答案:A解析:8.[单选题]相互关联的数据资产标准,应确保()。
数据资产标准存在冲突或衔接中断时,后序环节应遵循和适应前序环节的要求,变更相应数据资产标准。
A)连接B)配合C)衔接和匹配D)连接和配合答案:C解析:9.[单选题]固体半导体摄像机所使用的固体摄像元件为( )。
自适应贪婪算法在Web服务查询优化上的应用
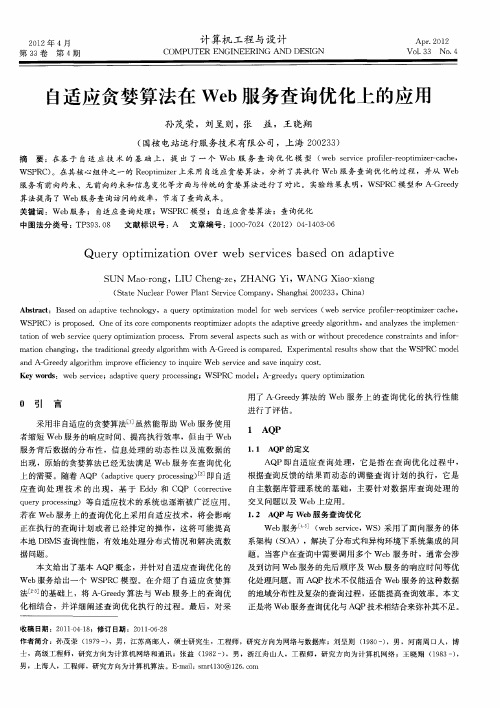
关键 词 :We 服务 ;自适应查询处理 ;WS R b P C模 型 ;自适应贪婪 算法;查询优化
中 图 法分 类 号 :T 3 3 0 文 献 标 识 号 : P 9 .8 A 文 章 编 号 : 0 07 2 (0 2 41 0—6 1 0—0 4 2 1 )0 —4 30
a d A- e d lo ih i r v fiin y t n u r e e v c n a e i q i o t n Gr e y ag r m t mp o ee f e c o i q ieW b s r iea d s v u r c s. c n y
0 引 言
采用非 自适应 的贪婪算法l 虽然能帮 助 We _ 1 ] b服务使用 者缩短 We b服务 的响应时 间、提高执行 效率 ,但 由于 We b 服务背后 数据 的分布性 ,信息处 理 的动 态性 以及流 数据 的 出现 ,原始的贪婪算法 已经无法 满足 We b服务在 查询优化
q eypoes g u r rcsi )等 自适应技术 的系统也逐渐被广 泛应用 。 n 若在 We b服务上 的查询优化上采用 自适 应技术 ,将 会影 响
正 在 执行 的查 询 计 划 或 者 已 经 排 定 的 操 作 , 这 将 可 能 提 高
We 服务[ ( e evc, )采用 了面 向服 务的体 b 4 w bsri WS e 系架构 ( O S A),解决 了分布式和异构环境下系统集成的问 题 。当客户在查询 中需要调用 多个 We b服务时 ,通常会涉 及到访问 We b服务的先后 顺序及 We b服务 的响应时间等优 化处理问题 。而 AQ P技术不仅能适合 We b服务的这种数据 的地域分布.及复杂的查询过程 ,还能提高查 询效 率。本文 『 生
一种结构化P2P网络中的动态协作缓存策略
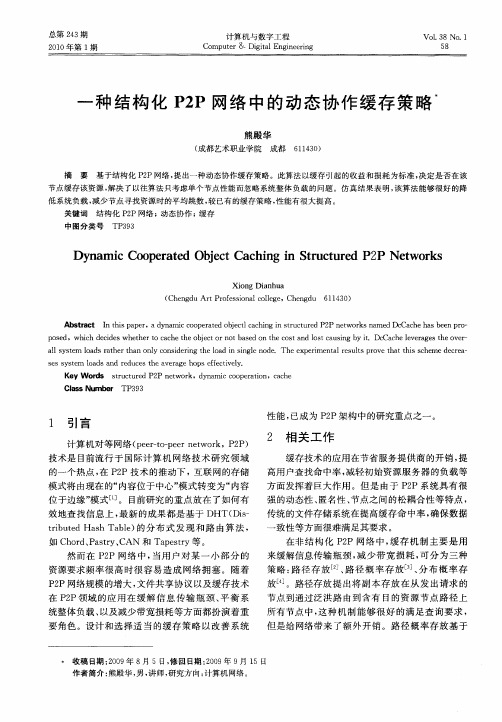
致性 等方 面很难 满 足其要 求 。 在 非 结 构 化 P P 网络 中 , 存 机 制 主 要 是 用 2 缓
然而 在 P P网 络 中 , 2 当用 户 对 某 一 小 部 分 的
来 缓解 信息 传输 瓶 颈 , 少带 宽 损 耗 , 减 可分 为 三 种 络 , 2 提出一种 动态协作缓存策略 。此算法 以缓存引起 的收益 和损耗为标 准 , 决定是否 在该
节点缓存该资源 , 决了以往 算法 只考 虑单个节 点性 能而忽 略系统 整体负载 的问题 。仿真结果表 明 , 解 该算法能够很好 的降 低 系统 负载 , 减少节点寻找资源时的平均跳数 , 已有 的缓存策略 , 能有很 大提高。 较 性
( e g u Ar o e so a o lg ,Ch n d 6 1 3 ) Ch n d tPr f s i n lc l e e egu 1 4 0
A s r c I i p p r ad n mi c o eae b et c c i tu t rdP P n t r s a dDc ah a e np o b ta t nt s a e , y a c o p rtdo jcl a hn i sr cu e 2 e h gn wok me C c eh s e r — n b p s d whc eie eh r occ ete be t r o a e nt ec s a dls c u igb .D C c el e a e e v r o e , ihd c s d wh t e h jc o t s do t n t a s yi c ah v rg s h e— t a h o n b h o o n t e t o
《2024年深度强化学习综述》范文

《深度强化学习综述》篇一一、引言深度强化学习(Deep Reinforcement Learning,简称DRL)是机器学习与强化学习相结合的产物,通过模拟人与环境交互的方式,实现了在复杂的动态环境中学习最优决策的策略。
深度强化学习的发展将人工智能领域向前推进了一大步,并引起了国内外研究者的广泛关注。
本文将对深度强化学习的原理、算法、应用等方面进行综述。
二、深度强化学习原理深度强化学习结合了深度学习和强化学习的优点,利用深度神经网络来表征状态和动作的价值函数,通过强化学习算法来优化这些价值函数,进而实现决策过程。
在深度强化学习中,智能体通过与环境的交互,逐渐学习到如何在给定状态下选择动作以最大化累积奖励。
这一过程主要包括感知、决策、执行三个环节。
三、深度强化学习算法深度强化学习的算法种类繁多,各具特色。
其中,最具代表性的算法包括基于值函数的Q-Learning、SARSA等,以及基于策略的Policy Gradient方法。
近年来,结合了深度学习和强化学习的优势的模型如Actor-Critic、Deep Q-Network(DQN)等算法受到了广泛关注。
这些算法在处理复杂问题时表现出了强大的能力。
四、深度强化学习应用深度强化学习在各个领域都有广泛的应用。
在游戏领域,AlphaGo等智能体通过深度强化学习算法,在围棋等游戏中取得了超越人类的成绩。
在机器人控制领域,深度强化学习可以帮助机器人通过与环境交互,学习到如何完成各种任务。
此外,在自动驾驶、医疗诊断、金融预测等领域,深度强化学习也展现出了巨大的潜力。
五、深度强化学习的挑战与展望尽管深度强化学习取得了显著的成果,但仍面临诸多挑战。
首先,如何设计有效的神经网络结构以更好地表征状态和动作的价值函数是一个重要的问题。
其次,在实际应用中,如何处理大规模的数据和复杂的交互过程也是一个难点。
此外,目前大多数深度强化学习算法仍依赖于大量的试错过程来优化策略,如何降低试错成本也是研究的一个重要方向。
SDN网络中基于深度强化学习的动态路由算法
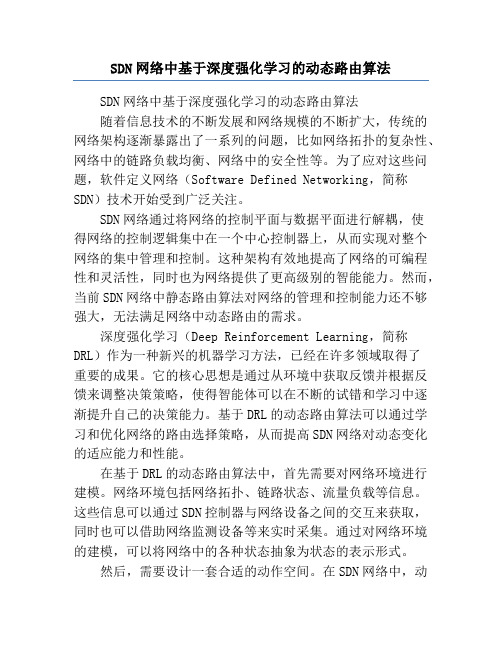
SDN网络中基于深度强化学习的动态路由算法SDN网络中基于深度强化学习的动态路由算法随着信息技术的不断发展和网络规模的不断扩大,传统的网络架构逐渐暴露出了一系列的问题,比如网络拓扑的复杂性、网络中的链路负载均衡、网络中的安全性等。
为了应对这些问题,软件定义网络(Software Defined Networking,简称SDN)技术开始受到广泛关注。
SDN网络通过将网络的控制平面与数据平面进行解耦,使得网络的控制逻辑集中在一个中心控制器上,从而实现对整个网络的集中管理和控制。
这种架构有效地提高了网络的可编程性和灵活性,同时也为网络提供了更高级别的智能能力。
然而,当前SDN网络中静态路由算法对网络的管理和控制能力还不够强大,无法满足网络中动态路由的需求。
深度强化学习(Deep Reinforcement Learning,简称DRL)作为一种新兴的机器学习方法,已经在许多领域取得了重要的成果。
它的核心思想是通过从环境中获取反馈并根据反馈来调整决策策略,使得智能体可以在不断的试错和学习中逐渐提升自己的决策能力。
基于DRL的动态路由算法可以通过学习和优化网络的路由选择策略,从而提高SDN网络对动态变化的适应能力和性能。
在基于DRL的动态路由算法中,首先需要对网络环境进行建模。
网络环境包括网络拓扑、链路状态、流量负载等信息。
这些信息可以通过SDN控制器与网络设备之间的交互来获取,同时也可以借助网络监测设备等来实时采集。
通过对网络环境的建模,可以将网络中的各种状态抽象为状态的表示形式。
然后,需要设计一套合适的动作空间。
在SDN网络中,动作空间可以包括路由路径的选择、链路的控制及流量的调度等。
通过将这些动作进行抽象和编码,可以使得智能体在选择动作时有更高的灵活性和选择性。
接下来,需要建立适当的奖励机制。
奖励机制通过对网络的性能评价和目标的设定来提供反馈信号,以引导智能体在学习和决策过程中逐步优化自身的行为。
如何在强化学习算法中处理长时间依赖问题(四)
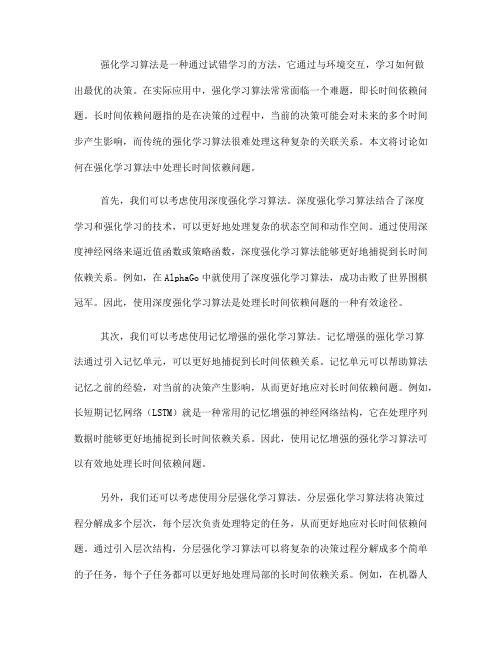
强化学习算法是一种通过试错学习的方法,它通过与环境交互,学习如何做出最优的决策。
在实际应用中,强化学习算法常常面临一个难题,即长时间依赖问题。
长时间依赖问题指的是在决策的过程中,当前的决策可能会对未来的多个时间步产生影响,而传统的强化学习算法很难处理这种复杂的关联关系。
本文将讨论如何在强化学习算法中处理长时间依赖问题。
首先,我们可以考虑使用深度强化学习算法。
深度强化学习算法结合了深度学习和强化学习的技术,可以更好地处理复杂的状态空间和动作空间。
通过使用深度神经网络来逼近值函数或策略函数,深度强化学习算法能够更好地捕捉到长时间依赖关系。
例如,在AlphaGo中就使用了深度强化学习算法,成功击败了世界围棋冠军。
因此,使用深度强化学习算法是处理长时间依赖问题的一种有效途径。
其次,我们可以考虑使用记忆增强的强化学习算法。
记忆增强的强化学习算法通过引入记忆单元,可以更好地捕捉到长时间依赖关系。
记忆单元可以帮助算法记忆之前的经验,对当前的决策产生影响,从而更好地应对长时间依赖问题。
例如,长短期记忆网络(LSTM)就是一种常用的记忆增强的神经网络结构,它在处理序列数据时能够更好地捕捉到长时间依赖关系。
因此,使用记忆增强的强化学习算法可以有效地处理长时间依赖问题。
另外,我们还可以考虑使用分层强化学习算法。
分层强化学习算法将决策过程分解成多个层次,每个层次负责处理特定的任务,从而更好地应对长时间依赖问题。
通过引入层次结构,分层强化学习算法可以将复杂的决策过程分解成多个简单的子任务,每个子任务都可以更好地处理局部的长时间依赖关系。
例如,在机器人控制领域,分层强化学习算法已经取得了一些成功的应用,能够更好地处理长时间依赖问题。
因此,使用分层强化学习算法也是处理长时间依赖问题的一种有效途径。
最后,我们还可以考虑使用基于奖励的强化学习算法。
基于奖励的强化学习算法将奖励信号作为学习的驱动力,可以更好地引导算法学习长时间依赖关系。
An Evalulation of the Pool Maintenance Overhead in Reliable Server Pooling Systems
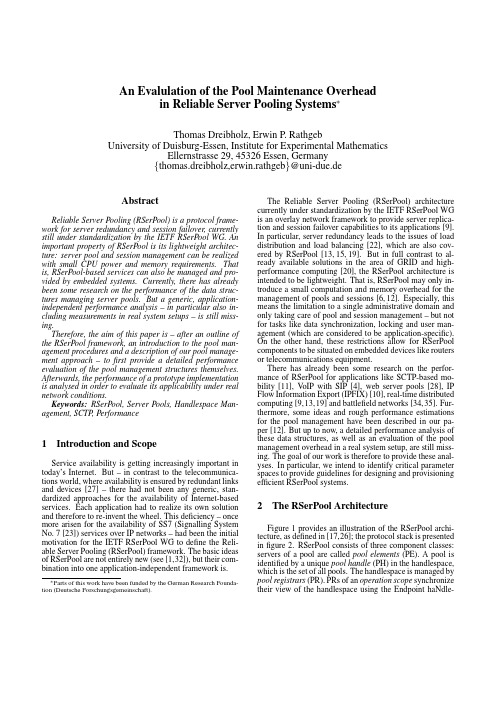
An Evalulation of the Pool Maintenance Overhead in Reliable Server Pooling Systems∗Thomas Dreibholz,Erwin P.RathgebUniversity of Duisburg-Essen,Institute for Experimental Mathematics Ellernstrasse29,45326Essen,Germany{thomas.dreibholz,erwin.rathgeb}@uni-due.deAbstractReliable Server Pooling(RSerPool)is a protocol frame-work for server redundancy and session failover,currently still under standardization by the IETF RSerPool WG.An important property of RSerPool is its lightweight architec-ture:server pool and session management can be realized with small CPU power and memory requirements.That is,RSerPool-based services can also be managed and pro-vided by embedded systems.Currently,there has already been some research on the performance of the data struc-tures managing server pools.But a generic,application-independent performance analysis–in particular also in-cluding measurements in real system setups–is still miss-ing.Therefore,the aim of this paper is–after an outline of the RSerPool framework,an introduction to the pool man-agement procedures and a description of our pool manage-ment approach–tofirst provide a detailed performance evaluation of the pool management structures themselves. Afterwards,the performance of a prototype implementation is analysed in order to evaluate its applicability under real network conditions.Keywords:RSerPool,Server Pools,Handlespace Man-agement,SCTP,Performance1Introduction and ScopeService availability is getting increasingly important in today’s Internet.But–in contrast to the telecommunica-tions world,where availability is ensured by redundant links and devices[27]–there had not been any generic,stan-dardized approaches for the availability of Internet-based services.Each application had to realize its own solution and therefore to re-invent the wheel.This deficiency–once more arisen for the availability of SS7(Signalling System No.7[23])services over IP networks–had been the initial motivation for the IETF RSerPool WG to define the Reli-able Server Pooling(RSerPool)framework.The basic ideas of RSerPool are not entirely new(see[1,32]),but their com-bination into one application-independent framework is.∗Parts of this work have been funded by the German Research Founda-tion(Deutsche Forschungsgemeinschaft).The Reliable Server Pooling(RSerPool)architecture currently under standardization by the IETF RSerPool WG is an overlay network framework to provide server replica-tion and session failover capabilities to its applications[9]. In particular,server redundancy leads to the issues of load distribution and load balancing[22],which are also cov-ered by RSerPool[13,15,19].But in full contrast to al-ready available solutions in the area of GRID and high-performance computing[20],the RSerPool architecture is intended to be lightweight.That is,RSerPool may only in-troduce a small computation and memory overhead for the management of pools and sessions[6,12].Especially,this means the limitation to a single administrative domain and only taking care of pool and session management–but not for tasks like data synchronization,locking and user man-agement(which are considered to be application-specific). On the other hand,these restrictions allow for RSerPool components to be situated on embedded devices like routers or telecommunications equipment.There has already been some research on the perfor-mance of RSerPool for applications like SCTP-based mo-bility[11],V oIP with SIP[4],web server pools[28],IP Flow Information Export(IPFIX)[10],real-time distributed computing[9,13,19]and battlefield networks[34,35].Fur-thermore,some ideas and rough performance estimations for the pool management have been described in our pa-per[12].But up to now,a detailed performance analysis of these data structures,as well as an evaluation of the pool management overhead in a real system setup,are still miss-ing.The goal of our work is therefore to provide these anal-yses.In particular,we intend to identify critical parameter spaces to provide guidelines for designing and provisioning efficient RSerPool systems.2The RSerPool ArchitectureFigure1provides an illustration of the RSerPool archi-tecture,as defined in[17,26];the protocol stack is presented infigure2.RSerPool consists of three component classes: servers of a pool are called pool elements(PE).A pool is identified by a unique pool handle(PH)in the handlespace, which is the set of all pools.The handlespace is managed by pool registrars(PR).PRs of an operation scope synchronize their view of the handlespace using the Endpoint haNdle-Figure1.The RSerPoolArchitectureFigure2.The RSerPool Protocol Stackspace Redundancy Protocol(ENRP[36]).In the operation scope,each PR is identified by a PR ID.An operation scope has a limited range,e.g.a company or organization;RSer-Pool does not intend to scale to the whole Internet.Never-theless,it is assumed that PEs can be distributed globally, for their service to survive localized disasters[16].A PE can register into a pool at an arbitrary PR of the operation scope,using the Aggregate Server Access Proto-col(ASAP[30]).In its pool,the PE will be identified by a random32-bit identifier which is denoted as PE ID.The PR chosen for registration becomes the Home-PR(PR-H) of the PE and is in particular also responsible for moni-toring the PE’s health by endpoint keep-alive messages.If not acknowledged,the PE is assumed to be dead and re-moved from the handlespace.Furthermore,PUs may re-port unreachable PEs;if a certain threshold of such reports is reached,a PR may also remove the corresponding PE. The PE failure detection mechanism of a PU is application-specific.A non-PR-H only sets a lifetime expiration timer for each PE(owned and monitored by another PR).If not updated by its PR-H in time,a PE is simply removed from the local handlespace.A client is called pool user(PU)in RSerPool terminol-ogy.To use the service of a pool given by its PH,a PU requests a PE selection–which is called handle resolution –from an arbitrary PR of the operation scope,again us-ing ASAP[30].The PR selects the requested list of PE identities using a pool-specific selection rule,called pool policy.The maximum number of selected entries per re-quest is defined by the constant MaxHResItems.Adaptive and non-adaptive pool policies are defined in[33];for a de-tailed discussion of these policies,see[13,15,19,37,38]. Relevant for this paper are the non-adaptive policies Round Robin(RR)and Random(RAND)and the adaptive policy Least Used(LU).LU selects the least-used PE,according to up-to-date load information;the actual definition of load is application-specific.Round robin selection is applied among multiple least-loaded PEs[12].The ASAP protocol also provides an optional Session Layer between a PU and a PE.That is,a PU establishes a logical session with a pool;ASAP takes care of the trans-port connection establishment,for the connection monitor-ing and for triggering a failover to a new PE in case of a fail-ure(see[5,14]).All associations among the three RSerPool component types(see alsofigure2)are usually based on the Stream Control Transmission Protocol(SCTP[29]),which in particular allows for path multi-homing(see[24,25]for details).3The Handlespace Management Approach 3.1RequirementsThe challenge of the handlespace management is to ful-fil two important properties,with particular regard of the “lightweight”requirement of the RSerPool architecture: (1)Server pools may get large(up to many thousands of PEs[8])and(2)A handlespace may contain various pools, each one may use a different policy for server selection[15] (and new applications may even introduce further poli-cies[16,19]).Clearly,in order to keep such a handlespace maintainable,it is necessary to use an unified storage struc-ture(which is usable for all policies)and realize it in an effi-cient way.Furthermore,the handlespace data structure has to support the following six operations:(1)Registration de-notes the registration of a new PE.(2)Deregistration means the removal of a PE entry.(3)Re-Registration is an infor-mation update for an exiting PE entry.In particular,a re-registration is necessary to update the policy information of an adaptive policy(e.g.the load state for LU[13]).(4)Han-dle Resolution denotes a PE selection operation.(5)Timer denotes scheduling and expiry of a handlespace timer.For a PR-H,this means scheduling a keep-alive transmission time,its timeout,scheduling a timeout for the keep-alive and cancelling it(on acknowledgement reception).For a non-PR-H,it denotes the scheduling of a registration’s life-time expiration and its cancellation(for an update).(6)Syn-chronization is the step-wise traversal of the complete hand-lespace,in order to obtain a block-wise copy for another PR.3.2Policy RealizationOn the topic of supporting different policies,we have already proposed in[12]to realize the handlespace in form of multiple sets(as illustrated infigure3):a handlespace is simply a set of pools(Pools Set);each pool contains a set of PE references sorted by PE ID(Index Set)and a second set of these references sorted by a policy-specific sorting order(Selection Set).In order to realize different policies,it is simply necessary to specify a sorting order for the Selection Set,as well as a selection procedure(which is usually to take thefirst PE).Upon selection of a PE entry,its position in the Selection Set is updated.In[12],we have already shown the scalability of this approach for a specific example application scenario.How-ever,a performance analysis for a broader parameter range has still been missing.Furthermore,our handlespace man-agement approach had to be extended by more features, which are described in the following.3.3Timer ScheduleScheduling and expiration of timers for PE entries is an additional task of the handlespace management.There are three types of timers:a keep-alive transmission timer sched-ules the transmission of an ASAP keep-alive to a PE;the keep-alive timeout timer schedules the timeout for the PE’s answer.A lifetime expiry timer schedules the expiration of a PE entry on a non-PR-H.An important observation for these three timers is that at any given time exactly one of them is scheduled for each PE.That is,each PE entry only has to contain the type of the timer and the expiration time stamp.Then,the timer schedule is simply another set of PE entries(sorted by time stamp,of course),as shown in figure3.3.4Checksum and Ownership SetThe ENRP protocol takes care of the handlespace syn-chronization.In order to detect discrepancies in the hand-lespace views of different PRs,each PR calculates a check-sum of its own PE entries(i.e.the PEs for which it is in the role of a PR-H).These checksums can be transmitted to other PRs,which can compare the value expected from their own handlespace view with the announced value.In case of a difference,a synchronization is necessary.The checksum algorithm used by ENRP is the16-bit Internet Checksum[3],which allows for incremental updates[9].The synchronization procedure requires to traverse all PE entries belonging to a certain PR.This functionality can be realized by introducing the so called Ownership Set–containing the PE references sorted by PR-H(seefigure3).Figure4.The Measurement Setup4The Measurement SetupIn[12],the pool management workload of a PR has al-ready been examined for different implementation strate-gies of the Set datatype–but only for a very specific setup.4.1Data Structure PerformanceHowever,a detailed analysis of the handlespace opera-tions throughput is still missing.Therefore,this will be thefirst part of this paper.Our program for the corre-sponding measurements simply performs as much opera-tions of the requested type as possible,in the pool built up in advance.Since registrations and deregistrations can-not be examined separately(the pool would either grow or shrink),these operations are examined combinedly:a Registration/Deregistration operation simply performs the deregistration of a randomly selected element if the pool has the configured size;otherwise,a new PE is registered. The system used for the performance measurements uses a 1.3GHz AMD Athlon CPU–which has been state of the art in early2001(i.e.almost seven years ago)and whose performance seems to be realistic for upcoming router or embedded device generations(which could host a PR ser-vice).All measurements are repeated18times in order to provide statistical accuracy.4.2Real System PerformanceWhile the operations throughput is useful to estimate the scalability of the handlespace management,the resulting question is clearly how a real system performs.In order to evaluate such a system,i.e.including real components, protocol stacks and network overhead,we have set up a lab scenario as shown infigure4:it consists of a set of10PCs (each having a2.4GHz Pentium IV CPU and1GB of mem-ory)connected by a gigabit switch to a Linux-based router. Two PRs(using the same CPU as for the data structure per-formance evaluation,see subsection4.1)are connected to the router by Gigabit Ethernet.On each of the hosts,a con-figurable number of test PEs,PUs and PRs can be started.All systems run Kubuntu Linux6.10“Edgy Eft”,us-ing kernel2.6.17-11and the kernel SCTP module providedby the distribution.Our RSerPool implementation RSP-LIB[7,9,18],version2.2.0has been installed on all ma-chines.Each measurement run is repeated12times toachieve statistical accuracy.GNU R has been used for the statistical post-processing of our results–including the computation of95%confi-dence intervals–and plotting.All results plots show the average values and their confidence intervals.5Performance AnalysisOur performance evaluation is subdivided into two parts. Thefirst part in subsection5.1provides a performance anal-ysis of the handlespace management structure itself and constitutes the foundation of the real system evaluation in subsection5.2.5.1Data Structure PerformanceThe most important operation for the PE side is the regis-tration/deregistration(see subsection3.1)at the PR.In[12], it has already been shown that deterministic policies can lead to systematic insertion and removal operations in the Selection Set(see subsection3.2).On the other hand,ran-domized policies are not affected.Therefore,only a bal-anced tree structure is appropriate to base the Set datatype on.We have examined the scalability on the number of PEs for the two state-of-the-art representations of this datatype: the red-black tree[21](a deterministic approach)and the treap[2](a randomized approach).The left-hand side offigure5shows the throughput of registration/deregistration operations per PE and second for both tree structures and classes of policies.While the per-formance difference between the two policy types is small, the treap has a slightly lower performance:using a deter-ministically balanced tree is–despite the greater complex-ity of the insertion and removal algorithms[21]–the faster solution.For a pool of20,000PEs,it would be possible to register or deregister each PE about2times per second (red-black tree).Clearly,this is more than sufficient in realistic scenar-ios.But while the frequency of registration/deregistration operations(i.e.actual insertions of new or removals of ex-isting PEs)is assumed to be rare,a re-registration(i.e.a registration update)of a PE occurs frequently,in particu-lar if the policy is dynamic.For a dynamic policy(e.g. LU),the position of the PE entry within the Selection Set changes(see also subsection3.2).In order to show the im-pact on the reregistration operations performance,the right-hand side offigure5presents the reregistrations throughput per PE and second.For the adaptive policy(here:LU),each reregistration updates the load value with a random value. As expected,a significant difference between adaptive and non-adaptive policies is shown:for20,000PEs,the non-adaptive policy still achieves a throughput of about5op-erations per PE and second(red-black tree),while it sinks to only about3in the adaptive case.That is,care has to be taken of the application behaviour–which actually has to decide when the policy information needs to be updated!Again,the performance for using a red-black tree is slightly better than using a treap.The throughput of timer operations is depicted on the left-hand side offigure6.Clearly,the two extreme cases for this operation are0%and100%of owned PEs.Therefore, the results of these two settings for both tree implementa-tions are shown.However,the difference keeps very small: re-scheduling a timer is quite inexpensive–the CPU’s cache helps to quickly re-insert the updated structure as described in subsection3.3.As already expected,the performance for a red-black tree is slightly better than for a treap.Handle resolution is the operation relevant for the PUs. Its performance is influenced by two factors:MaxHRe-sItems and the type of policy–randomized or determin-istic.For a randomized policy,it is necessary to move down the Selection Set tree(whose depth is O(log n)–n number of PEs–for red-black tree and treap)in order to obtain a random PE[12]–for each of the MaxHResItems entries. Deterministic policies,on the other hand,simply allow for taking a complete chain of PE entries from the list(since their order is deterministic and therefore already defined by the sorting order,see subsection3.2),i.e.the overall run-time is O(1)instead.The throughput of handle resolution operations per PE and second is depicted on the right-hand side offigure6. Clearly,it can be observed that the higher MaxHResItems, the lower the throughput:it sinks from13at MaxHRe-sItems h=1to about7.5at h=3for10,000PEs(determinis-tic policy,red-black tree).Furthermore,the performance for a randomized policy is clearly lower:7at h=1vs.about4 at h=3for10,000PEs(red-black tree).Again,the perfor-mance for the treap is somewhat lower than for the red-black tree.In a real system,the frequency of handle reso-lutions strongly depends on the application’s PU workload. Having a PU with a high handle resolution frequency(e.g.a web proxy like[28]),it is possible to apply a handle resolu-tion cache at the PU[13].Furthermore,the handle resolu-tion operation has an advantage over the previously exam-ined operations:it can be performed independently of other PRs.That is,in case of a high handle resolution workload, the PUs could be distributed among multiple PRs.The last operation is the synchronization,which only oc-curs when PRs detect an inconsistency or on PR startup. That is,the operation is quite rare(e.g.up to a few times per day only).However,the actual performance for a pool of30,000PEs allows for more than100operations per sec-ond,which is by orders of magnitude more than sufficient. Therefore,a plot has been omitted.5.2Real System PerformanceWhile our RSerPool handlespace management approach –based on red-black trees–handles pools of10,000and more PEs,pools of up to a few hundreds of PEs seem to be most realistic for the application cases of RSerPool.There-fore,the following measurements focus on smaller pools, but with a high PR request frequency in order to fathom the limits.050001000015000200002500051015202530Number of Pool Elements [1]R e g i s t r a t i o n O p e r a t i o n s p e r P E a n d S e c o n d [1/P E *s ]50001000015000200002500051015202530Number of Pool Elements [1]R e −R e g i s t r a t i o n O p e r a t i o n s p e r P E a n d S e c o n d [1/P E *s]Figure 5.The Scalability of the Registration/Deregistration and Re-Registration Operations050001000015000200002500051015202530Number of Pool Elements [1]T i m e r O p e r a t i o n s p e r P E a n d S e c o n d [1/P E *s ]50001000015000200002500051015202530Number of Pool Elements [1]H a n d l e R e s o l u t i o n O p e r a t i o n s p e r P E a n d S e c o n d [1/P E *s ]Figure 6.The Scalability of the Timer Handling and Handle Resolution Operations5.3Pool Elements ScalabilityIn order to show the scalability on PEs,the number of PEs has been varied.The pool is using the RR pol-icy (i.e.deterministic)and an inter-reregistration time be-tween 250ms and 1000ms (such high rates may occur for dynamic policies).All ASAP (re-)registrations are per-formed on PR #1(see figure 4),PR #2is synchronized by ENRP only.That is,we have used the worst case here.The CPU utilization of PR #1and PR #2are shown on the left-hand side of figure 7.Randomized policy results have been omitted,since the results do not differ significantly (see also subsection 5.1).Clearly,the workload on PR #1is highest:it not only has to handle up to 3,000simultaneous SCTP associations to PEs (for ASAP),but also has to send out an ENRP update to the other PR on every update of a PE entry.This leads to a load of about 90%for 2,000PEs at an inter-reregistration time of a =250ms.Extending this time to a =1000ms,it isalready possible to manage 3,000PEs at a load of only about 25%.Obviously,the workload of PR #2is significantly lower:it only has to maintain a single SCTP association to PR #1to obtain the handlespace data.This results in a load of only about 15%for 2,000PEs at a =250ms,and about 25%for 3,000PEs at a =1000ms.It is therefore a clear recom-mendation to try to distribute the load among the PRs of the operation scope.In reality,this can be achieved using the automatic configuration feature of RSerPool [34].However,care has to be taken of redundancy:in case of PR failure(s),there must be a sufficient number of other PRs!But what about the costs of the ENRP synchronization among PRs?5.4Registrars ScalabilityIn order to show the scalability on the number of PRs,we have again used PR #1for the ASAP associations and PR #2for ENRP synchronization only (as shown in figure 4).Fur-Pool Elements e [1]Registrar's PerspectiveRegistrars G [1]Provider's PerspectiveFigure 7.Registrar CPU Utilization for Pool Maintenancether PRs have been started on the other PCs (since only the utilizations of PR #1and PR #2are relevant).For our measurement,we have used a pool of 1,000PEs and inter-reregistration times of a =250ms to a =1000ms.The CPU utilization results for PR #1and PR #2are presented on the right-hand side of figure 7.Clearly,the number of PRs does not significantly af-fect PR #2.While it has to maintain an association with each other PR of the operation scope,the actual workload –which remains constant –is only transported via the as-sociation with PR #1.On the other hand,the utilization for PR #1is significantly increased with the number of PRs,in particular if the inter-reregistration time is small:e.g.from about 20%for a single PR to slightly more than 60%for 6PRs (at a =250ms).The bottleneck in this case is the in-terface between userland application (i.e.the PR)and the kernel’s SCTP API.For each PR,a separate ENRP mes-sage has to be passed to the kernel’s SCTP API.Clearly,the context switching and memory copying for this opera-tion is time-consuming,while the actual message transport (IP packets via Ethernet interface)is quite efficient (a recent system can transport hundreds of thousands of packets per second).The analysis of the described userland/kernel bottleneck has led to the suggestion of a SCTP API extension:the SCTP SENDALL option (see subsection 5.2.2of [31]).Us-ing this option,a message to all PRs is passed to the kernel only once –and sent via all PR associations.But although the new option is already a part of the SCTP API standards document [31],it is not implemented for the current Linux kernel (version 2.6.20)yet.Therefore,a performance eval-uation using this option has to be part of future work.In summary,using a reasonably small number of PRs (e.g.two or three are usually sufficient to achieve redun-dancy),the ENRP overhead remains in an acceptable range –with room for future improvement on the SCTP layer.5.5Pool Users ScalabilityFinally,we have evaluated the scalability on the num-ber of PUs for handle resolution operations using two PRs.Again,we have observed the CPU utilization of PR #1and PR #2(see figure 4)for a pool of 1,000PEs using deter-ministic (solid lines)and randomized policies (dotted lines),an inter-reregistration time of 1000ms and inter-handle-resolution times between 100ms and 500ms.For the first measurement,we have used PR #1for both,registrations and handle resolutions (left-hand side),while we have put the burden of handle resolutions on PR #2for the second measurement (right-hand side).Clearly,if using PR #1for all operations,PR #2only has to synchronize and therefore its load keeps constant.But nevertheless,the CPU load of PR #1only slightly ex-ceeds 25%for 2,000PUs and a inter-handle-resolution time of 500ms.For a higher handle resolution rate,however,the CPU utilization quickly grows:at 100ms,there is already a load of more than 80%for 1,000PUs.The performance difference between the two types of policies is small –even at 2,000PEs,the CPU utilization of a randomized policy is only by less than 5%higher (see subsection 5.1).That is,compared to the protocol overhead,the pool maintenance effort is small for this number of PEs.So,with regard to these results,it is obviously a good idea to split up the workload of registration management and handle resolutions among the PRs.Therefore,PR #2in the second measurement (right-hand side of figure 4)is re-sponsible for all handle resolutions.Clearly,the system per-formance gets better now:at a CPU utilization below 80%(PR #2),it is now possible to serve 1,500PUs with a handle-resolution rate of only 100ms –at a workload of about 10%for PR #1.Splitting up the workload of both operations be-tween the two PRs would clearly result in an even better performance.However,a redundant system should always be provisioned for the worst case –which is a failure of n −1of the n PRs.That is,the sum of the workloads of both PRs must remain significantly lower than 100%!Pool Users u [1]Registrar's Perspective using PR #1Pool Users u [1]Registrar's Perspective using PR #2Figure 8.Registrar CPU Utilization for Handle Resolution5.6Results SummaryIn summary,our handlespace performance analysis has shown that our approach of reducing the handlespace man-agement to the storage of sets and operations on these sets is efficient if using a red-black tree to actually realize the sets.Critical operations are the re-registration (which may occur very frequently for adaptive policies)and the handle resolution.But in our real system performance analysis,we have shown that even a low-performance CPU is able to handle scenarios of significantly more than 1,000PEs and PUs.As general recommendation,it is useful to dis-tribute the PEs und PUs to different PRs of the operation scope to achieve the highest performance.However,care has to be taken of sufficient PR redundancy to cope with PR failures.Depending on the inter-reregistration and handle resolution frequency,also much larger scenarios are possi-ble.A room for further performance improvement will be the SCTP SENDALL option of the SCTP stack,which will be realized in future SCTP implementations.6ConclusionsThe analyses of this paper have shown that our hand-lespace realization is efficient:using a red-black tree as base structure to store the handlespace content,all hand-lespace operations can be reduced to the management of balanced trees.The performance of this approach is suffi-cient to maintain handlespaces of many thousands of PEs –even on a low-performance CPU being realistic for upcom-ing routers and embedded systems.In the second part of this paper,we have also proven that our approach is applicable and efficient in reality:a system based on the same CPU is also capable of handling the ASAP/ENRP protocol overhead and the maintenance of SCTP associations.As part of our future research,we are going to further evaluate our approach for certain RSerPool-based applica-tion scenarios.Such real-world scenarios set requirementson pool size and policy type as well as on re-registration and handle resolution frequency.In particular,we intend to es-timate a lower threshold for the CPU performance needed to handle these application scenarios.This also includes tests with our implementation on Linux-based embedded systems.References[1]L.Alvisi,T.C.Bressoud,A.El-Khashab,K.Marzullo,andD.Zagorodnov.Wrapping Server-Side TCP to Mask Con-nection Failures.In Proceedings of the IEEE Infocom 2001,volume 1,pages 329–337,Anchorage,Alaska/U.S.A.,Apr.2001.ISBN 0-7803-7016-3.[2] C.Aragon and R.Seidel.Randomized search trees.In Pro-ceedings of the 30th IEEE Symposium on Foundations of Computer Science ,pages 540–545,Oct.1989.[3]R.Braden,D.Borman,and puting theInternet Checksum.Standards Track RFC 1071,IETF,Sept.1988.[4]P.Conrad,A.Jungmaier,C.Ross,W.-C.Sim,and M.T¨u xen.Reliable IP Telephony Applications with SIP using RSer-Pool.In Proceedings of the State Coverage Initiatives,Mobile/Wireless Computing and Communication Systems II ,volume X,Orlando,Florida/U.S.A.,July 2002.ISBN 980-07-8150-1.[5]T.Dreibholz.An Efficient Approach for State Sharing inServer Pools.In Proceedings of the 27th IEEE Local Com-puter Networks Conference (LCN),pages 348–352,Tampa,Florida/U.S.A.,Oct.2002.ISBN 0-7695-1591-6.[6]T.Dreibholz.Policy Management in the Reliable ServerPooling Architecture.In Proceedings of the Multi-Service Networks Conference (MSN,Cosener’s),Abingdon,Ox-fordshire/United Kingdom,July 2004.[7]T.Dreibholz.Das rsplib–Projekt –Hochverf¨u gbarkeit mitReliable Server Pooling.In Proceedings of the LinuxTag ,Karlsruhe/Germany,June 2005.[8]T.Dreibholz.Applicability of Reliable Server Pool-ing for Real-Time Distributed Computing.Internet-DraftVersion 03,IETF,Individual Submission,June 2007.draft-dreibholz-rserpool-applic-distcomp-03.txt,work in progress.。
贝尔曼方程在神经元网络中的应用

贝尔曼方程在神经元网络中的应用贝尔曼方程是强化学习的核心概念之一,它被用来计算一系列动作的奖励值,以帮助决策制定者做出最优选择。
但是,它不仅仅被应用于强化学习领域,还可以被应用于神经元网络中,从而提高神经元网络的效率和精度。
神经元网络是由神经元构成的网络,它可以用于模拟人脑的工作原理,从而实现人工智能、机器学习等领域的任务。
神经元网络包含输入层、隐藏层以及输出层,它们之间的连接都有不同的权重和偏置,而这些权重和偏置可以通过训练来得出。
然而,在神经元网络中,数据的处理过程可能会出现误差和噪声,从而影响神经元网络的精度和效率。
而贝尔曼方程可以被用来解决这个问题。
在神经元网络中,贝尔曼方程可以被用来计算预测值和真实值之间的误差,并通过误差来调整网络中的权重和偏置。
例如,在神经元网络中,利用贝尔曼方程来计算reward的值,可以帮助网络更准确地预测reward在神经元网络中的值,从而提高神经元网络的效率和精度。
除此之外,贝尔曼方程可以被用来优化神经元网络中的目标函数,从而进一步提高神经元网络的性能。
目标函数是一种衡量神经元网络性能的指标,它会随着权重和偏置的变化而变化。
而利用贝尔曼方程来优化目标函数,则将神经元网络的性能最大化。
因此,在神经元网络中,贝尔曼方程被广泛应用于人工智能、机器学习等领域。
它可以帮助我们更准确地预测数据,并优化神经元网络的效率和精度。
相信未来会有更多的研究者应用它在神经元网络领域中,带来更多的突破和进步。
绝对延迟保证在Web应用服务器数据库连接池中的实现

作者简介 :吕健波( 90 ) 男, 17 - , 吉林人 , 讲师 , 博士 , 主要研 究方向为 We o 、 bGS等 (i bl@ht alcn) bQ S We I jno a u om i o ;戴冠 中( 9 7 ) 男, 海 . 13 一 , 上
人, 教授 , 导, 博 主要研究方向为控 制理论 与控制工程、 复杂网络 中的控制 问题 ; 慕德俊 (9 3 ) 男, 16 一 , 山东人 , 教授 , 博导, 主要研 究方向为并行计 算、
W e i sc mp s d o re t r d a c i cu e, h sp p rp o o e n mp e ne b ou e d ly g a a te i h aa b st o o e fa t e — e e r ht tr t i a e r p sd a d i lme td a s l t ea u r ne n te d t— e h i e
关键词 :We 用服务 器 ;反馈控 制 ;绝对延 迟保 证 ;系统辨 识 ; 据库 连接池 b应 数
中 图分 类号 :T 3 3 P 9
文献标 志码 :A
文章 编号 :10 —6 5 2 1 )5 13 —4 0 1 39 (0 2 0 — 8 8 0
di1 .9 9 ji n 1 0 — 6 5 2 1 .5 0 3 o:0 3 6 7.s .0 1 39 . 0 2 0 .6 s
m ae ln a i - v fa tmo l ft t i e rtme-n a n deso i i he DBCP t r u h s tm de t c to n esg e hea out ea ua a e o r l h o g yse i ni a in a d d in d t bs l e d ly g rnte c nto- i f - l r I mplme t d alt o o nt fco e lo si e lDBCP o h o a e a lc to ev r T s eulss o e . ti e n e l hec mp ne so ls d—o p n a ra frt e T mc tW b pp iain s r e . e tr s t h w
人工神经网络与神经网络优化算法

其中P为样本数,t j, p 为第p个样本的第j个输
出分量。
感知器网络
1、感知器模型 2、学习训练算法 3、学习算法的收敛性 4.例题
感知器神经元模型
感知器模型如图Fig2.2.1 I/O关系
n
y wipi bi
i 1
y {10
y0 y0
图2.2.1
单层感知器模型如图2.2.2
定义加权系数
10.1 人工神经网络与神经网络优化算法
③第 l 1层第 i个单元到第个单元的权值表为
; l1,l ij
④第 l 层(l >0)第 j 个(j >0)神经元的
输入定义为 , 输出定义 Nl1
x
l j
y l 1,l ij
l 1 i
为
yLeabharlann l jf (xlj )
, 其中 i0 f (•)为隐单元激励函数,
人工神经网络与神经网络优化算法
自20世纪80年代中期以来, 世界上许多国 家掀起了神经网络的研究热潮, 可以说神 经网络已成为国际上的一个研究热点。
1.构成
生物神经网
枝蔓(Dendrite)
胞体(Soma)
轴突(Axon) 胞体(Soma)
2.工作过程
突触(Synapse)
生物神经网
3.六个基本特征: 1)神经元及其联接; 2)神经元之间的联接强度决定信号传递的强
函数的饱和值为0和1。
4.S形函数
o
a+b
c=a+b/2
(0,c)
net
a
2.2.3 M-P模型
McCulloch—Pitts(M—P)模型, 也称为处理单元(PE)
x1 w1
abstractvalueadaptingcache 的 get 和 lookup -回复

abstractvalueadaptingcache 的get 和lookup -回复题目: abstractvalueadaptingcache 的get 和lookup 方法详解摘要: abstractvalueadaptingcache是在缓存系统中常用的一种机制,它通过get和lookup方法来实现缓存数据的获取和查找。
本文将详细介绍abstractvalueadaptingcache的概念、用途以及如何使用get和lookup 方法。
第一部分: 概念介绍abstractvalueadaptingcache是一种在缓存系统中经常使用的机制。
它允许将缓存与外部数据源进行适配,以便在缓存系统中实现更高效的数据访问。
abstractvalueadaptingcache的主要功能是在缓存数据的获取和查找时进行适配转换。
第二部分: 使用get方法获取缓存数据在abstractvalueadaptingcache中,get方法是用来获取缓存数据的主要方式。
当我们需要从缓存中获取数据时,可以使用get方法来实现。
get方法的使用步骤如下:1. 调用get方法并传入需要获取数据的Key。
例如: cache.get(key)。
2. 系统会首先在缓存中查找对应的数据。
3. 如果找到了匹配的数据,则直接返回该数据。
4. 如果没有找到匹配的数据,则尝试从外部数据源中获取数据。
5. 如果从外部数据源中获取到了数据,则将数据放入缓存中,并返回。
get方法的一些注意事项:1. get方法返回的数据类型通常是Object,需要根据实际情况进行类型转换。
2. 在使用get方法时,需要注意缓存的过期时间和淘汰策略,以保证数据的准确性和及时性。
3. 在高并发情况下,可能会出现多个线程同时进行数据获取的情况,需要注意并发安全性。
第三部分: 使用lookup方法查找缓存数据除了get方法,abstractvalueadaptingcache还提供了lookup方法来实现缓存数据的查找。
基于深度强化学习的网络资源分配与调度
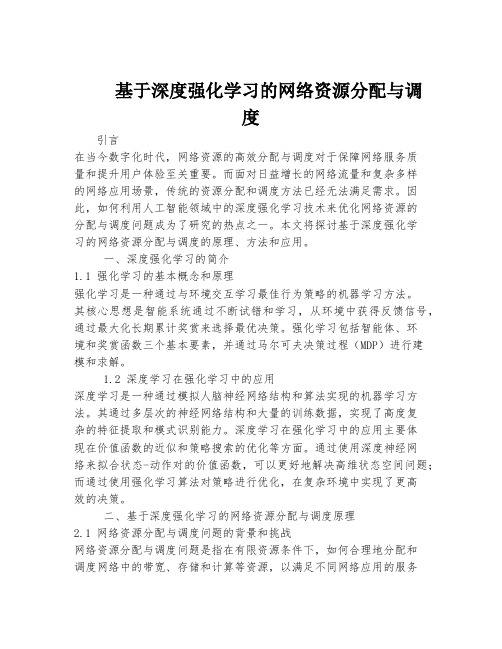
基于深度强化学习的网络资源分配与调度引言在当今数字化时代,网络资源的高效分配与调度对于保障网络服务质量和提升用户体验至关重要。
而面对日益增长的网络流量和复杂多样的网络应用场景,传统的资源分配和调度方法已经无法满足需求。
因此,如何利用人工智能领域中的深度强化学习技术来优化网络资源的分配与调度问题成为了研究的热点之一。
本文将探讨基于深度强化学习的网络资源分配与调度的原理、方法和应用。
一、深度强化学习的简介1.1 强化学习的基本概念和原理强化学习是一种通过与环境交互学习最佳行为策略的机器学习方法。
其核心思想是智能系统通过不断试错和学习,从环境中获得反馈信号,通过最大化长期累计奖赏来选择最优决策。
强化学习包括智能体、环境和奖赏函数三个基本要素,并通过马尔可夫决策过程(MDP)进行建模和求解。
1.2 深度学习在强化学习中的应用深度学习是一种通过模拟人脑神经网络结构和算法实现的机器学习方法。
其通过多层次的神经网络结构和大量的训练数据,实现了高度复杂的特征提取和模式识别能力。
深度学习在强化学习中的应用主要体现在价值函数的近似和策略搜索的优化等方面。
通过使用深度神经网络来拟合状态-动作对的价值函数,可以更好地解决高维状态空间问题;而通过使用强化学习算法对策略进行优化,在复杂环境中实现了更高效的决策。
二、基于深度强化学习的网络资源分配与调度原理2.1 网络资源分配与调度问题的背景和挑战网络资源分配与调度问题是指在有限资源条件下,如何合理地分配和调度网络中的带宽、存储和计算等资源,以满足不同网络应用的服务质量要求。
随着网络规模的不断扩大和用户对高带宽、低延迟的需求日益增长,资源分配和调度问题变得愈发复杂和困难。
2.2 深度强化学习在网络资源分配与调度中的应用深度强化学习方法在网络资源分配与调度问题中的应用主要可以分为以下几个方面:(1)基于深度Q网络(Deep Q-Network,DQN)的带宽分配:通过使用DQN算法,将网络的带宽分配问题建模为MDP,通过不断学习和优化网络的带宽分配策略,实现带宽的智能调度和分配。
基于自动机器学习的云平台动态资源调度研究

基于自动机器学习的云平台动态资源调度研究1. 前言随着云计算技术的快速发展,云平台已经成为了企业和个人使用计算资源的主要方式。
云平台上资源的动态调度一直是一个重要的问题。
资源调度不当会导致资源的浪费,也会影响用户对云平台的体验。
如何利用自动机器学习技术来实现云平台资源的动态调度,一直是一个备受关注的研究领域。
2. 云平台动态资源调度的挑战在云平台上进行资源调度面临着多个挑战。
云平台上有大量的虚拟化资源,包括虚拟机、存储和网络资源等。
这些资源的需求和使用是动态变化的,需要根据实时的需求来进行调度。
云平台上的工作负载也是非常复杂的,包括了各种类型的应用程序和服务,它们的性能和资源需求各不相同。
由于云平台上资源的使用是多租户的,资源调度需要考虑多个用户之间的需求和冲突。
面对这些挑战,传统的资源调度方法往往难以满足实时的需求。
研究人员开始探索利用自动机器学习技术来实现云平台资源的动态调度。
3. 基于自动机器学习的云平台资源调度方法利用自动机器学习技术来进行云平台资源调度,主要包括以下几个步骤:3.1 数据采集和特征提取需要对云平台上的资源和工作负载进行数据采集,包括CPU、内存、网络等资源的使用情况,以及各种应用程序和服务的性能和资源需求。
然后,需要对这些数据进行特征提取,找到对资源调度有意义的特征。
3.2 模型训练与选择接下来,需要利用机器学习算法来训练资源调度模型。
常用的机器学习算法包括决策树、支持向量机、神经网络等。
在训练模型时,需要考虑到资源调度的实时性和准确性。
还需要根据不同的场景选择合适的机器学习模型。
3.3 模型部署与优化一旦训练好了机器学习模型,就需要将其部署到云平台上。
在部署的过程中,需要考虑到模型的实时性和可靠性。
还需要对模型进行优化,以确保其在实际调度中能够取得良好的效果。
4. 研究进展与挑战目前,已经有一些研究工作利用自动机器学习技术来进行云平台资源调度。
这些工作在一定程度上取得了一些成果,但也面临着一些挑战。
池化的参考文献
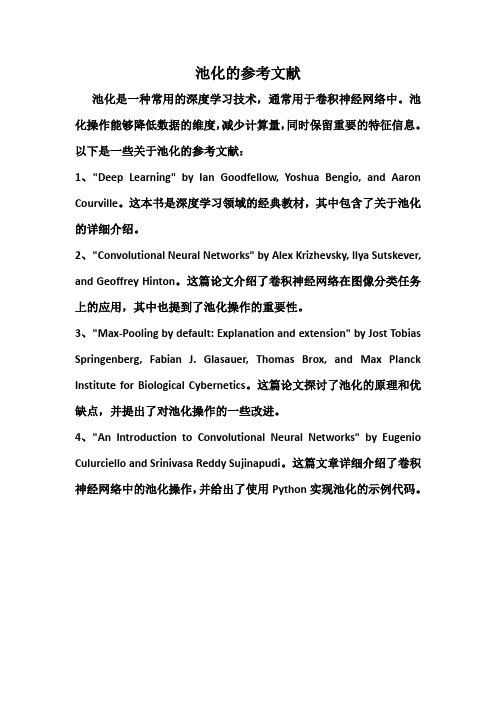
池化的参考文献
池化是一种常用的深度学习技术,通常用于卷积神经网络中。
池化操作能够降低数据的维度,减少计算量,同时保留重要的特征信息。
以下是一些关于池化的参考文献:
1、"Deep Learning" by Ian Goodfellow, Yoshua Bengio, and Aaron Courville。
这本书是深度学习领域的经典教材,其中包含了关于池化的详细介绍。
2、"Convolutional Neural Networks" by Alex Krizhevsky, Ilya Sutskever, and Geoffrey Hinton。
这篇论文介绍了卷积神经网络在图像分类任务上的应用,其中也提到了池化操作的重要性。
3、"Max-Pooling by default: Explanation and extension" by Jost Tobias Springenberg, Fabian J. Glasauer, Thomas Brox, and Max Planck Institute for Biological Cybernetics。
这篇论文探讨了池化的原理和优缺点,并提出了对池化操作的一些改进。
4、"An Introduction to Convolutional Neural Networks" by Eugenio Culurciello and Srinivasa Reddy Sujinapudi。
这篇文章详细介绍了卷积神经网络中的池化操作,并给出了使用Python实现池化的示例代码。
支撑性服务自动化

⽀撑性服务⾃动化连载传送门:什么是云原⽣?云原⽣设计理念.NET 微服务谈到云原⽣,绕不开“容器化”Backing services云原⽣系统依赖于许多不同的辅助资源,例如数据存储、消息队列、监视和⾝份服务。
这些服务统称为⽀撑性服务。
下图显⽰了云原⽣系统使⽤的许多常见⽀撑性服务⽀撑性服务帮助实现了“⼗⼆要素应⽤”中的Statelessness原则要素6提到:“每个微服务应在独⽴隔离的进程中执⾏,将所需状态信息作为外部⽀撑性服务,例如分布式缓存或数据存储”最佳实践是将⽀撑性服务视为附加资源,并使⽤外部挂载的⽅式将配置(URL和凭据)动态绑定到微服务。
要素4指出: “⽀撑性服务“应通过可寻址的URL公开,这样做解耦了将资源与应⽤”要素3指出: “将配置信息从微服务中移出并外挂”Stateless和⽀撑性服务,这样松散的设计使你可以将⼀项⽀撑性服务换成另⼀项⽀撑性服务,或将您的代码移⾄其他公有云,⽽⽆需更改主线服务代码。
⽀撑性服务将在第5章“云原⽣数据模式”和第4章“云原⽣通信模式”中详细讨论。
⾃动化如你所见,云原⽣依赖(微服务、容器和现代设计理念)来实现速度和敏捷性。
但是,那只是故事的⼀部分,你如何配置运⾏这些系统的云环境?你如何快速部署应⽤程序功能和更新?被⼴泛认可的作法是基础设施即代码(IaC)借助IaC,你可以⾃动化平台配置和应⽤程序部署,你将诸如测试和版本控制之类的软件⼯程实践应⽤于您的DevOps实践。
你的基础架构和部署是⾃动化,⼀致且可重复的。
Automating infrastructure在底层,IaC是幂等的,这意味着你可以⼀遍⼜⼀遍地运⾏相同的脚本,⽽不会产⽣副作⽤。
如果团队需要进⾏更改,可以编辑并重新运⾏脚本,(仅)需要更新的资源受到影响。
在《基础架构即代码》⼀书中,作者Sam Guckenheimer指出:“实施IaC的团队可以⼤规模、快速、稳定地交付。
团队不⽤⼿动配置环境,通过代码表⽰的所需环境状态,来增强交付预期。
移动数据库中基于Agent的缓存一致性策略
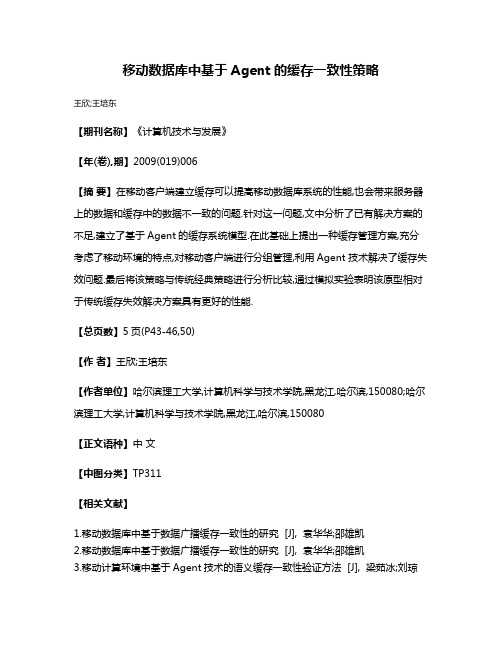
移动数据库中基于Agent的缓存一致性策略
王欣;王培东
【期刊名称】《计算机技术与发展》
【年(卷),期】2009(019)006
【摘要】在移动客户端建立缓存可以提高移动数据库系统的性能,也会带来服务器上的数据和缓存中的数据不一致的问题.针对这一问题,文中分析了已有解决方案的不足,建立了基于Agent的缓存系统模型.在此基础上提出一种缓存管理方案,充分考虑了移动环境的特点,对移动客户端进行分组管理,利用Agent 技术解决了缓存失效问题.最后将该策略与传统经典策略进行分析比较,通过模拟实验表明该原型相对于传统缓存失效解决方案具有更好的性能.
【总页数】5页(P43-46,50)
【作者】王欣;王培东
【作者单位】哈尔滨理工大学,计算机科学与技术学院,黑龙江,哈尔滨,150080;哈尔滨理工大学,计算机科学与技术学院,黑龙江,哈尔滨,150080
【正文语种】中文
【中图分类】TP311
【相关文献】
1.移动数据库中基于数据广播缓存一致性的研究 [J], 袁华华;邵雄凯
2.移动数据库中基于数据广播缓存一致性的研究 [J], 袁华华;邵雄凯
3.移动计算环境中基于Agent技术的语义缓存一致性验证方法 [J], 梁茹冰;刘琼
4.基于广播的移动客户机缓存数据一致性策略 [J], 刘涛;蒋外文;胡景蕙;宋富强
5.移动云环境中基于Agent的缓存一致性维护策略 [J], 张以利;杨万扣
因版权原因,仅展示原文概要,查看原文内容请购买。
基于聚类的非一致性数据库查询重写

基于聚类的非一致性数据库查询重写
谢东;杨路明;蒲保兴;刘波
【期刊名称】《小型微型计算机系统》
【年(卷),期】2007(28)12
【摘要】在非一致性数据库上,以元组匹配技术所产生的聚类和概率数据库的元组概率为基础,提出了可信聚类概率和可重写查询判断方法.考虑了最普通的IC情况(key-to-key和 nonkey-to-key),给出了无连接和有连接的查询重写方法.连接查询重写方法缩小了用于连接的中间结果集中可信聚类的元组数量,有效地提高了查询性能.实验使用TPC-H决策支持基准的数据和查询进行性能研究,分析了聚类基数和数据库尺寸等相关因素的影响,结果显示方法是有效的.
【总页数】4页(P2199-2202)
【作者】谢东;杨路明;蒲保兴;刘波
【作者单位】中南大学,信息科学与工程学院,湖南,长沙,410083;中南大学,信息科学与工程学院,湖南,长沙,410083;中南大学,信息科学与工程学院,湖南,长沙,410083;中南大学,信息科学与工程学院,湖南,长沙,410083
【正文语种】中文
【中图分类】TP311
【相关文献】
1.非一致性数据库的概率查询重写 [J], 谢东;杨路明;蒲保兴;刘波
2.基于tableau结点封闭值的非一致性数据库开放分支修复方法 [J], 高龙;刘全;傅
启明;李娇
3.非一致性数据库的多关系聚集查询重写 [J], 张华兵;杨路明;谢东;王佳宜
4.基于聚类的非一致性数据库聚集查询重写 [J], 谢东;杨路明;蒲保兴;刘波
5.基于范围语义的非一致性数据库聚集查询 [J], 谢东;吴敏
因版权原因,仅展示原文概要,查看原文内容请购买。
- 1、下载文档前请自行甄别文档内容的完整性,平台不提供额外的编辑、内容补充、找答案等附加服务。
- 2、"仅部分预览"的文档,不可在线预览部分如存在完整性等问题,可反馈申请退款(可完整预览的文档不适用该条件!)。
- 3、如文档侵犯您的权益,请联系客服反馈,我们会尽快为您处理(人工客服工作时间:9:00-18:30)。
Implementing the Reliable Server PoolingFrameworkThomas DreibholzUniversity of Duisburg-EssenEllernstrasse2945326Essen,Germany Email:dreibh@exp-math.uni-essen.de Telephone:+49201183-7637Fax:+49201183-7373Erwin P.RathgebUniversity of Duisburg-EssenEllernstrasse2945326Essen,Germany Email:rathgeb@exp-math.uni-essen.de Telephone:+49201183-7670Fax:+49201183-7373Abstract—The Reliable Server Pooling(RSerPool)pro-tocol suite currently under standardization by the IETF is designed to build systems providing highly available services by mechanisms and protocols for establishing,configuring, accessing and monitoring pools of server resources.But RSerPool is not only able to manage pools of redundant servers and facilitate service failover between servers:it also includes sophisticated mechanisms for server selections within the pools.These mechanisms make RSerPool useful for applications in load balancing and distributed computing scenarios.As part of our RSerPool research and to verify results of our simulation model in real-life scenarios,we have created a complete implementation prototype of the RSer-Pool framework.In this paper,we will give a detailed description of the concepts,ideas and realizations of our prototype.Furthermore,we will show performance issues raised by the management of large servers pools,as itis necessary for load balancing or distributed computing scenarios.We will explain the algorithms and data structures we designed to solve these challenges andfinally present a rough performance evaluation that verifies our concept.Keywords:Internet applications,IPv6deployment and applications,SS7,server poolsI.R ELIABLE S ERVER P OOLINGA.MotivationThe convergence of classical circuit-switched networks (i.e.PSTN/ISDN)and data networks(i.e.IP-based)is rapidly progressing.This implies that PSTN signalling via the SS7protocol is transported over IP networks. Since SS7signalling networks offer a very high degree of availability(e.g.at most10minutes downtime per year for any signalling relationship between two signalling endpoints;for more information see[1]),all links and components of the network devices must be redundant. When transporting signalling over IP networks,such redundancy concepts also have to be applied to achieve the required availability.Link redundancy in IP net-works is supported using the Stream Control Transmission Protocol(SCTP[2],[3],details follow in section II); redundancy of network device components is supported by the SGP/ASP(signalling gateway process/application server process)concept[1].However,this concept has some limitations:no support of dynamic addition and re-moval of components,limited ways of server selection,noFig.1.The RSerPool Architecturespecific failover procedures and inconsistent application to different SS7adaptation layers.B.IntroductionTo cope with the challenge of creating a unified, lightweight,real-time,scalable and extendable redun-dancy solution(see[4]for details),the IETF Reliable Server Pooling Working Group was founded to spec-ify and define the Reliable Server Pooling concept.An overview of the architecture currently under standardiza-tion and described by several Internet Drafts is shown in figure1.Multiple server elements providing the same service belong to a server pool to provide both redundancy and scalability.Server pools are identified by a unique ID called pool handle(PH)within the set of all server pools, the handlespace.A server in a pool is called a pool element(PE)of the respective pool.The handlespace is managed by redundant registrars(PR).The registrars synchronize their view of the handlespace using the End-point haNdlespace Redundancy Protocol(ENRP[5]).PRsFig.2.Registration and Monitoringannounce themselves using multicast mechanisms,i.e.it is not necessary(although possible)to pre-configure PR addresses into the components described in the following. PEs providing a specific service can register for a corresponding pool at an arbitrary PR using the Aggregate Server Access Protocol(ASAP[6])as shown infigure2. The home PR(PR-H)is the PR which was chosen by the PE for initial registration.It monitors the PE using SCTP heartbeats(layer4,not shown infigure;see section II)and ASAP Endpoint Keep Alives.RSerPool does not rely on the layer4heartbeat mechanism of SCTP here:the application itself could e.g.hang in an infinite loop while the system’s kernel is still responding to the SCTP ing additional keep alives above SCTP therefore improves the monitoring reliability. The frequency of monitoring messages depends on the availability requirements of the provided service.When a PE becomes unavailable,it is immediately removed from the handlespace by its home PR.A PE can also inten-tionally de-register from the handlespace by an ASAP de-registration allowing for dynamic reconfiguration of the server pools.PR failures are handled by requiring PEs to re-register regularly(and therefore choosing a new PR when necessary).Re-registration also makes it possible for the PEs to update their registration information(e.g. transport addresses or policy states).The home PR,which registers,re-registers or de-registers a PE,propagates this information to all other PRs via ENRP.Therefore,it is not necessary for the PE to use any specific PR.In case of a failure of its home PR,a PE can simply use another arbitrarily chosen one.C.Server SelectionWhen a client requests a service from a pool,itfirst asks an arbitrary PR to translate the pool handle to a list of PE identities selected by the pool’s selection policy(pool policy),e.g round robin or least used(we show examples in section V-B;the standards policies are defined in[7],a quantitative policy performance comparison can be found in[8]).The PU adds this list of PE identities to its local cache(denoted as PU-side cache) and again selects one entry from its cache by policy.To this selected PE,a connection is established,using the application’s protocol,to actually use the service.The client then becomes a pool user(PU)of the PE’s pool. It has to be emphasized,that there are two locations where a selection by pool policy is applied during this process:1)at the PR when compiling the list of PEs and2)in the local PU-side cache where the target PE isselected from the list.If the connection to the selected PE fails,e.g.due to overload or failure of the PE,the PU selects another PE (i.e.directly from cache or by asking a PRfirst)and tries again.The PU may report a PE failure to a PR,which may decide to remove this PE from the handlespace. D.Failover ProcedureRSerPool supports optional client-based state synchro-nization[9]for failover:a PE can store its current state with respect to a specific connection in a state cookie which is sent to the corresponding PU.When a failover to a new PE is necessary,the PU can send this state cookie to the new PE,which can then restore the state and resume service at this point.However,RSerPool is not restricted to client-based state synchronization;any other application-specific failover procedure can be used as well.E.The Protocol StackFigure3illustrates the RSerPool protocol stack.All components are based on SCTP over IPv4and/or IPv6. For the PR,the application layer consists of ENRP and ASAP.While ENRP provides handlespace redundancy between multiple PRs,ASAP is used for registration,re-registration,de-registration and monitoring of PEs as well as for handle resolutions and failure reports by PUs. Between a PU and PE,ASAP becomes a session layer protocol that provides the client-based state synchro-nization as described in section I-D.This session layer communication,called control channel,is multiplexed with the application’s protocol,called data channel,over the same SCTP association.Optionally,PRs can announce themselves via ASAP and ENRP via multicast so that other PRs,PEs and PUs may be fully auto-configuring.This functionality has been omitted in thefigure to enhance its readability.F.ApplicationsThe lightweight,real-time,scalable and extendable ar-chitecture of RSerPool is not only applicable to the trans-port of SS7-based telephony signalling;other applica-tion scenarios include reliable SIP-based telephony[10], mobility management[11]and the management of dis-tributed computing pools[12],[13].Finally,load balancing using RSerPool is currently under discussion by the IETF RSerPool Working Group: due to itsflexible server selection policies and pool man-agement functionalities,it has many similarities to loadFig.3.The RSerPool Protocol Stackbalancer protocols.A very common application for such load balancing systems is to distribute HTTP requests in web server farms.There is an ongoing effort to merge both the RSerPool framework and the Server/Application State Protocol (SASP [14],a contribution of IBM)for load balancers into one common architecture for highly-available server pool management and load distribution.II.T HE SCTP P ROTOCOLWhile the duty of RSerPool is to provide fault-tolerance against component failures,it relies on the SCTP transport protocol [2]to provide fault-tolerance against network failures.As explained in the introduction,SCTP allows multi-homing to fulfil the fault tolerance requirements of SS7.That is,two SCTP endpoints can be connected via two or more networks.When there are multiple disjoint paths between the two endpoints,SCTP can use another one when its primary path becomes unavailable.Such unavailability can occur by network component and link failures or simply due to long convergence times of inter-domain routing protocols (e.g.in the range of several minutes for BGP).SS7requires a failover time of at most 800ms and SCTP is able to satisfy this requirement [15];from an endpoint’s view,each destination address is considered as a possible path –denoted as SCTP path [2]–to transmit data over.SCTP uses path monitoring to check these paths for availability:in configurable intervals,SCTP sends control messages,called heartbeats ,over each possible path.The peer endpoint,when receiving such a heartbeat,acknowledges it by sending a heartbeat acknowledgement .Paths on which acknowledgements are received,are considered to be usable paths for data transport.When the actual data transport path (called primary path )becomes unavailable,a working one is selected and the data transmission is continued.The whole process of path monitoring and selection of a new primary path is transparent to the application layer.For details on the configuration of suitable heartbeat intervals and path selection parameters,see [15].SCTP has been designed to be independent of the underlying network layer protocol,i.e.it is not only possible to use IPv4and IPv6but also adapt it to other or future protocols.In the view of SCTP,network layerprotocols appear as SCTP paths to the multi-homing functionality.For example,an endpoint supports IPv4and IPv6and the peer endpoint is reachable via IPv4and IPv6.Then,an association between these endpoints has two SCTP paths:one via IPv4and one via IPv6.If there is a failure e.g.on the IPv4path,it is therefore still possible to use the IPv6path.Such multi-protocol setups are very likely in today’s networks,due to the growing IPv6deployment in formerly IPv4-only networks.In the area of telecommunications,associations are established for durations in the range of months or even years.Therefore,it has been necessary to define a dynamic address reconfiguration extension (abbreviated Add-IP ,see [16])allowing for the dynamic addition to and removal of transport addresses from an SCTP association without connection interruption.This especially allows interruption-free IPv6site renumbering,i.e.changing the address prefix on a provider change to keep BGP routing tables small or even add an additional provider for redundancy reasons.Furthermore,it even allows an association to be established in an IPv4-only network,being upgraded to IPv4+IPv6and finally turned into IPv6only –interruption-free and transparent to the upper layers.III.T HE RS ER P OOL APIThe programming API for RSerPool is currently ac-tively being discussed by the IETF RSerPool WG.It will consist of two styles:the basic mode and the enhanced mode .A.Basic Mode APIThe basic mode provides only the fundamental RSer-Pool function calls for PEs to register,re-register or de-register and for PUs to resolve a pool handle and select a PE by policy.All session layer functionalities between PE and PU –especially failure detection and failover –have to be provided by the application programs themselves.That is,a control channel is not supported here.The reason for having the basic mode API is to provide easy deployment of RSerPool functionality to existing applications,e.g.a FTP service application that supports download continuation using FTP’s reget functionality.An example for using the basic mode API can be found in[12].B.Enhanced Mode APIUnlike the basic mode API,the enhanced mode API offers a complete session layer between PE and PU, including optional failover handling using client-basedstate synchronization.That is,a PU establishes a session to a pool provid-ing its desired service.The session layer provided by the enhanced mode API transparently handles pool han-dle resolutions,PE selections,association establishments, failure detection on the association using SCTP heart-beats,selecting a new PE when the former one becomes unreachable and optionally failover handling using state cookies via the control channel.For the application itself, this session layer can be completely transparent1.In fact, the pool appears to the user as one highly available server. To provide easy adaptation of existing and new ap-plications to RSerPool’s session layer functionality,the API mimics the Unix socket API to provide session layer functionality.A pseudo-code example is shown in algo-rithm1:similarly to creating a TCP socket,connecting it to a remote server andfinally using the application’s protocol to do something,a RSerPool session is created, connected to a pool and the application’s protocol is used over the session.But unlike a simple TCP connection, RSerPool provides seamless service continuation in case of server failure–transparent to the application.The PE side of the enhanced mode API also looks similar to TCP-based servers,but instead of binding a socket to a port number,it is registered as PE under the service’s pool handle.Note,that applications do not have to care about any transport address when using the enhanced mode API.A PE is by default registered under all of its transport addresses–regardless of whether they are IPv4or IPv6. Furthermore,using the Add-IP[16]extension of SCTP as described in section II,transport addresses may change at runtime,e.g.due to IPv6prefix change.At the PU side, transport association management and therefore handling of addresses is completely transparent to the application layer.Currently,there are only two existing implementations of RSerPool:a closed source version by Motorola[17] and the authors’own GPL-licensed Open Source proto-type rsplib.This prototype will be explained in detail in the following section.IV.T HE rsplib P ROTOTYPEAs part of our RSerPool research and to verify the results of our simulation model[8],[18]in real-life sce-narios,we have created a complete implementation[12], [19]prototype of the RSerPool framework.It consists of a PR and a library–the rsplib–providing the PE and PU functionalities.Our implementation package,called the 1If the application uses a custom failover procedure,some interaction may berequired.Fig.4.The rsplibRegistrarFig.5.The rsplib PU/PE Libraryrsplib prototype,has been released[19]as Open Source under the GPL license.Elementary design criteria of our prototype have been platform independence and the support of both IPv4 and IPv6.To ensure platform independence,we have chosen C instead of C++as implementation language, because C is more common on exotic devices.Although currently only Linux,FreeBSD and Darwin(MacOS X) are supported by our prototype,our long-term goal is to make it also available on embedded devices like PDAs and smartphones.A short-term goal is to extend our support to the Windows and Solaris platforms.When we started the development of our prototype in2002,the only stable SCTP implementation on our three main platforms(Linux,FreeBSD,Darwin)has been our own Open Source userland SCTP implementation sctplib[20].Meanwhile,the native SCTP support of these platforms has improved so that we also support the built-in kernel SCTP of Linux,FreeBSD(KAME stack) and Darwin.All afore-mentioned SCTP implementations, including our own sctplib,support the Add-IP extension for dynamic address reconfiguration.The rsplib prototype is a complete implementation of RSerPool,also including the features being optional in the standards documents.In particular,we support both the basic and enhanced mode APIs,full auto-configuration by PR announcements via multicast–both,for ASAP and ENRP–and all optional policies defined in the draft[7]. This draft is one contribution,based on our RSerPool research on policy performance[8],and has become a working group draft of the IETF RSerPool WG.Algorithm1A PU Pseudo-Code Examplersp_connect(sd,"MyDownloadServerPool",...);rsp_write(sd,"GET MyMovie.mpeg HTTP/1.0\r\n\r\n"); while((length=rsp_read(sd,buffer,...))>0){ doSomething(buffer,length);}rsp_close(sd);The building blocks of the rsplib prototype are shown infigure4(registrar)andfigure5(PU/PE library).Both parts contain the Dispatcher component encapsulating the platform-dependent timer andfile/socket event manage-ment as well as thread-safety functionality.On top of this component,the registrar realizes the ASAP and ENRP protocols.Their functionality is controlled by the Reg-istrar Management,which consists of the binding layer between protocols and the registrar’s central component: the Handlespace Management.This component takes care of storing the handespace’s content and providing access functionality for both ASAP(registration,re-registration, deregistration and monitoring of PEs,handle resolutions for PUs)and ENRP(handlespace synchronization be-tween PRs).Authenticating and authorizing requests to the handlespace management is the duty of the Registrar Management.It also takes care of the optional transmis-sion of ASAP and ENRP announcements via multicast. For PUs and PEs,the Dispatcher is the foundation of the ASAP Instance component.The ASAP Instance consists of three sub-components:ASAP Protocol is the implementation of ASAP for communication to PRs and between PE and PU via the multiplexed control/data chan-nel.For creating and parsing ASAP messages,it contains the sub-components ASAP Creator and ASAP Parser.In the Registrar Table,addresses of usable PRs–either stat-ically configured or learned by the PRs’announcements via multicast–are managed.When communication to a PR is necessary,this component also takes care of establishing a connection to a PR.The last sub-component of the ASAP Instance is the ASAP Cache,i.e.the PU-side cache for handle resolutions.For the implementation,the data structures and algorithms necessary to manage the cache are equal to the PR’s handlespace management. Therefore,its code can be reused here.In an early version of our prototype,we realized the handlespace management using linear lists and provided only round robin as pool policy.This workedfine for simple lab scenarios;however,there has been a grow-ing demand to realize additional policies like random selection or least used for the research on load distribu-tion performance.Furthermore,pools of load balancing scenarios can become very large(hundreds of elements) and efficiency becomes crucial.Therefore,our simple approach became unsuitable and a more sophisticated handlespace management concept was necessary.We will explain our concept in the followingsection.Fig.6.Handlespace ManagementV.H ANDLESPACE M ANAGEMENTBefore we describe our implementation of the han-dlespace management,wefirst define it as abstract datatype:the handlespace is a set of n pools(n∈N), denoted by PH h1to h n.Each poolπcontains a non-empty set of PE entries,denoted by their PE IDiπk∈{0,...,232−1}⊂N0.A PE entry includes the PE’s policy information yπk(e.g. the PE’s load in case of LU policy)and the PE’s non-empty set of transport addresses aπk.The following operations must be possible on the handlespace datatype:1)Insertion,lookup and removal of pools by poolhandle;2)Insertion,lookup and removal of PEs within a poolby PE ID;3)Selection of PEs within a pool by policy;4)Traversal of the handlespace for ENRP synchro-nization purposes.Furthermore,it should be easily possible to add new selection policies for new applications.A.Implementing the HandlespaceImplementing the abstract handlespace datatype be-comes straightforward as illustrated infigure6:there is a set of pools sorted by pool handle and each pool contains two sets of PE references–thefirst set sorted by PE ID(solid line),the second set sorted by a sorting order defined by the pool’s policy(dotted line).We will explain later how to actually implement a set.A policy-specific selection procedure implements the selection of a PE.In the default case,this simply means to selectthefirst element from the set ordered by the sorting ing the structures above,it is only necessary to define a specific sorting order and selection procedure to implement a certain policy.Such definitions are the next step.B.Implementing PoliciesBefore we define sorting order and selection procedure for some important policies defined in[7],we introduce two helper constructs for simplification:For simplifying randomized selection,we define the following:to every PE entry i,a value v i∈R may be mapped.It has to be possible to request the sum(called value sum)V=iv iof all PE entries’values within the set.Then,randomized selection is possible by choosing a numberr∈R{0,...,V}⊂R.Since the set is ordered,r specifies the uniquely identifi-able PE entry j that satisfies the conditioni=1,...,j−1v i<r≤v j+i=1,...,j−1.Furthermore,to guarantee uniqueness of sorting orders, we add sequence numbers to pools and PE entries:each pool gets a pool sequence number and each PE a PE sequence number.Every time a PE entry i is inserted into the selection set or being selected,its PE sequence number seq i is set to the pool sequence number of its pool. Finally,this pool sequence number is then incremented by1.Now,we can define some example policies and show how the helper constructs are used:a)Round Robin:The only sorting key is the PE entries’sequence number in ascending order and the selection procedure is the default one,i.e.getting thefirst element of the set.An example is given in table I:the upper block shows the pool“Example”before a selection.A selection returns PE entry ID-#1(since it is thefirst entry of the set),its sequence number is set to the pool sequence number(4)and it is reinserted into the pool. Since it now has the highest sequence number,it is appended to the end of the set.Finally,the pool sequence number is incremented by one.A further selection will fetch PE ID-#2,then PE-ID-#3,again PE ID-#1and so on,providing the desired round robin behaviour.b)Weighted Random:Since random selection can-not take elements from the top of the selection set but has to use values v i and their sum V,it is only necessary to ensure that the sorting keys in the set are unique. Using the PE sequence number as sorting key ensures this property.For the weighted random policy,the value v i of PE entry i is set to the PE’s given weight constant. Table II shows an example:the pool consists of3PEs where PEs ID-#6and ID-#7have weight1(therefore v= 1).PE ID-#2has weight3(and therefore v=3)and PE ID-#8has weight2(and therefore v=2).The weight sum is thereforeV=1+2+3+1=7.For a selection,a random numberr∈R{0,...,7}⊂Ris chosen.Let r=5.75.In this case,only j=3satisfies the conditioni=1,...,j−1v i<5.75≤v j+i=1,...,j−1,that is1+3<5.75≤1+3+2.Then,the third(j-th)PE of the set is selected:PE ID-#8. Using an uniform distribution for choosing r,weighted random results in the desired behaviour of selecting PEs at a probability proportional to their weight constant.c)Least Used:Using the least used policy,each PE’s policy information specifies the current server load as value from0%to100%.Clearly,thefirst part of the sorting key is this load value in ascending order.The second part is the PE sequence number in ascending order. We use the default selection procedure,i.e.taking the set’sfirst element.Obviously,this will select the PE of the least load.And for the case that there are multiple PEs having the same least load,the PE sequence number as second part of the composite sorting key ensures round robin selection between these elements.Clearly,arbitrary other policies can be expressed through definition of a sorting order and a selection procedure.That is,our policy implementation concept of-fers a solid foundation for future and application-specific extensions.C.PerformanceAfter definition of data structure and policies,the only remaining question of handlespace management is how to implement the datatype for the required sets.The naive solution is to simply use a linear list.A more efficient solution may be to use a binary tree,a red-black tree[21] (balanced tree)or a treap[22](randomized tree).But does the effort for realistic pool sizes justify a more complicated structure?To answer this question,we made a rough performance evaluation of our handlespace management implementa-tion on an Athlon1.3GHz CPU.We have chosen this CPU since its power seems to be realistic for upcoming router CPU generations2–routers are devices on which a PR process could be started.For our handlespace performance evaluation,we are not interested in SCTP or network layer efficiency,therefore we omit it here. As test scenario,we assume two large pools,using the least used policy,in which we scale the average amount of PEs from1to1000.Since pools map to specific applications,it is realistic to assume a small amount 2The current Juniper ERX1400,a300,000US$router,only contains a Pentium-III at500MHzTABLE IR OUND R OBIN P OLICY E XAMPLEPool“Example”Policy RRseq=4Pool Element ID-#1seq=1Pool Element ID-#2seq=2Pool Element ID-#3seq=3Pool“Example”Policy RRseq=5Pool Element ID-#2seq=2Pool Element ID-#3seq=3Pool Element ID-#1seq=4TABLE IIW EIGHTED R ANDOM P OLICY E XAMPLEPool“Example”Policy WRR seq=5V=7PoolElementID-#7seq=1,v=1Pool Element ID-#2seq=2,v=3PoolElement ID-#8seq=3,v=2PoolElement ID-#6seq=4,v=1Fig.7.Performance(e.g.less than20).Furthermore,applications requiringsignificantly large pools are assumed to be rare(e.g.a web server farm or a distributed computing service).Therefore,two large pools seem to be realistic.We omitadding additional small pools(e.g.2to5PEs)here,sincethis would not significantly affect the results.Each PE is assumed to handle10PU requests/s(themore PEs,the more PU requests–adding servers onlymakes sense when there is more work to be done).Thatis,10handle resolutions per second and PE are requiredfrom the handlespace management.A PE stays registeredfor an average duration of30m(uniform distribution)andthen deregisters.During its runtime,a re-registration ismade every30s(default from[5]).When a PE is removed,a new PE is added to keep the average amount of PEsconstant.Synchronization(this means traversal of thehandlespace)is made every5minutes.The handlespaceis a priorifilled with the given amount of PEs;then,each test runs for10m.The more components are inthe scenario,the more handlespace operations have tobe executed.For statistical accuracy,each test has beenrepeated5times;the shown results are the average valuesand their95%confidence intervals,being computed by RProject.Figure7shows the CPU’s load as a fraction of the run-time(10m)required for handlespace operations in percentfor the implementation of a set by linear list,binary tree,red-black tree and treap.On the x-axis,the total amount ofPEs is shown(they divide up to the two pools).Obviously,balanced trees(red-black)and randomized trees(treap)。