Real-Time Constraints
Time Constraints Boost Popularity of Online Dating网上求爱日渐流行(双语阅读)

Time Constraints Boost Popularity of Online Dating网上求爱日渐流行Any lingering stigma about finding true love online seems to be fading,particularly among older adults, researchers fiound研究人员发现,在网络上寻找真爱似乎已经不再是什么令人羞愧的事,尤其是对于大龄成年人而言。
In a study of 175 newlywed couples scientists at Iowa State University said those who met through online dating agencies, or social networking sites, tended to be older than other couples who met through traditional ways offline'. They were also less likely to be marrying for the first time and had shorter courtshipsZ before tying the knot一18.5 months instead of 42 months.爱荷华州立大学的科学家在一项针对175对新婚夫妇开展的研究中发现,他们当中,通过在线交友网站或社交网站结识的夫妇通常要比那些通过传统的“线下”方式结识的夫妇年龄大。
同样,通过网络所结成的伴侣,他们是初婚的几率较小,而且在结婚前谈恋爱的时间也较短,仅为18.5个月,而对于传统婚姻模式,谈恋爱的时间是42个月。
"In many cases, there are real structural forces that encourage the support and use of these technologies,”said Alicia Cast, an associate professor of sociology at the university.爱荷华州立大学社会学副教授艾丽西亚·卡斯特说:“在很多情况下,真实存在的结构性力量促使了人们对这些技术的支持和使用。
Autodesk Nastran 2023 参考手册说明书
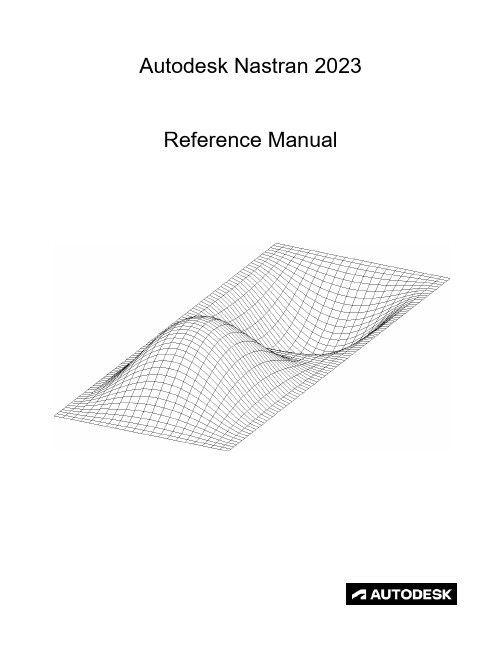
FILESPEC ............................................................................................................................................................ 13
DISPFILE ............................................................................................................................................................. 11
File Management Directives – Output File Specifications: .............................................................................. 5
BULKDATAFILE .................................................................................................................................................... 7
Incentive-compatible, asymmetric-information, real
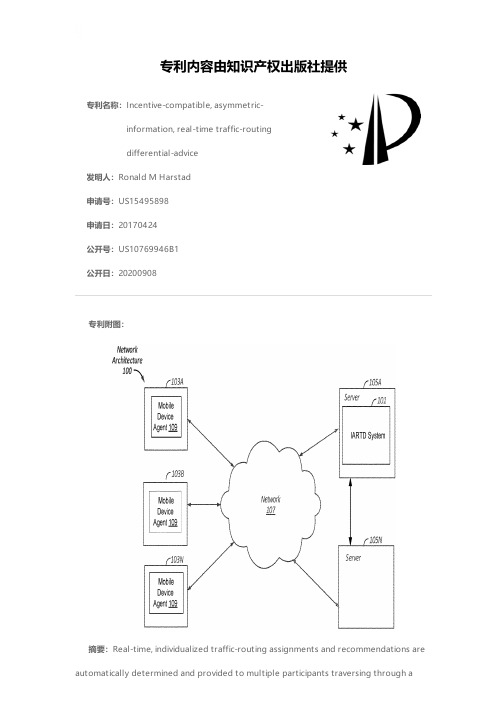
专利名称:Incentive-compatible, asymmetric-information, real-time traffic-routingdifferential-advice发明人:Ronald M Harstad申请号:US15495898申请日:20170424公开号:US10769946B1公开日:20200908专利内容由知识产权出版社提供专利附图:摘要:Real-time, individualized traffic-routing assignments and recommendations are automatically determined and provided to multiple participants traversing through aspecific area in association with a given event associated with a particular organization. A set of rules is applied to minimize the time to traverse through the area in association with the event, summed over the multiple participants, accounting for physical and incentive-compatibility constraints. An initial assignment is determined for each participant, based on the rules. Each initial assignment includes a departure time and an initial route for the participant. Updated information is received, such as real-time traffic information. Real-time recommendations are determined for participants, based on the rules accounting for the updated real-time data. Recommendations include suggestions to deviate from initial assignments or previous recommendations, based on the updated information. The initial assignments and real-time recommendations are provided to the corresponding participants, for example by communicating with their mobile devices.申请人:Ronald M Harstad地址:Columbia MO US国籍:US代理机构:Patent Law Works LLP更多信息请下载全文后查看。
ISE时序约束笔记
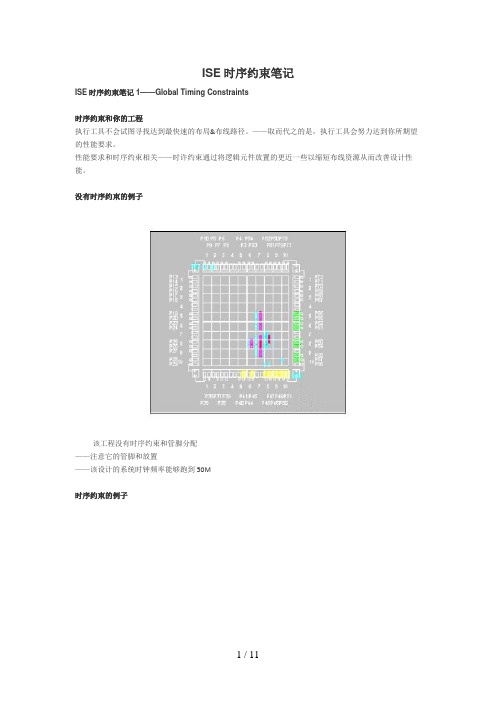
ISE时序约束笔记ISE时序约束笔记1——Global Timing Constraints时序约束和你的工程执行工具不会试图寻找达到最快速的布局&布线路径。
——取而代之的是,执行工具会努力达到你所期望的性能要求。
性能要求和时序约束相关——时许约束通过将逻辑元件放置的更近一些以缩短布线资源从而改善设计性能。
没有时序约束的例子该工程没有时序约束和管脚分配——注意它的管脚和放置——该设计的系统时钟频率能够跑到50M时序约束的例子和上面是相同的一个设计,但是加入了3个全局时序约束。
——它最高能跑到60M的系统时钟频率——注意它大部分的逻辑的布局更靠近器件边沿其相应管脚的位置更多关于时序约束时序约束应该用于界定设计的性能目标1.太紧的约束将会延长编译时间2.不现实的约束可能导致执行工具罢工3.查看综合报告或者映射后静态时序报告以决定你的约束是否现实执行后,查看布局布线后静态时序报告以决定是否你的性能要求达到了——如果约束要求没有达到,查看时序报告寻找原因。
路径终点有两种类型的路径终点:1.I/O pads2.同步单元(触发器,锁存器,RAMs)时序约束的两个步骤:1.路径终点生产groups(顾名思义就是进行分组)2.指点不同groups之间的时序要求全局约束使用默认的路径终点groups——即所有的触发器、I/O pads等ISE时序约束笔记2——Global Timing Constraints问题思考单一的全局约束可以覆盖多延时路径如果箭头是待约束路径,那么什么是路径终点呢?所有的寄存器是否有一些共同点呢?问题解答什么是路径终点呢?——FLOP1,FLOP2,FLOP3,FLOP4,FLOP5。
所有的寄存器是否有一些共同点呢?——它们共享一个时钟信号,约束这个网络的时序可以同时覆盖约束这些相关寄存器间的延时路径。
周期约束周期约束覆盖由参考网络钟控的的同步单元之间的路径延时。
周期约束不覆盖的路径有:input pads到output pads之间的路径(纯组合逻辑路径),input pads到同步单元之间的路径,同步单元到output pads之间的路径。
Path Planning Robot Navigation

Path Planning Robot NavigationPath planning in robot navigation is a critical aspect of robotics that involves determining the best route for a robot to take in order to reach its destination. This process is essential for ensuring efficient and safe movement of robots in various environments, such as warehouses, hospitals, and even on the streets. However, path planning comes with its own set of challenges and considerations, including the need to balance between optimality, feasibility, and real-time constraints. In this response, we will explore the complexities of path planning in robot navigation, as well as the various approaches and technologies used to address these challenges.One of the key considerations in path planning for robot navigation is the need to balance between optimality and feasibility. On one hand, the goal is to find the most optimal path that minimizes the distance traveled or maximizes the robot's efficiency. On the other hand, the path must also be feasible, taking into account obstacles, dynamic environments, and real-time constraints. This balance is crucial for ensuring that the robot can navigate safely and efficiently in its environment. Achieving this balance often requires the use of advanced algorithms and technologies that can dynamically adapt to changing conditions and constraints.Another important consideration in path planning for robot navigation is the need to address dynamic environments. In real-world scenarios, the environment in which a robot operates is constantly changing, with obstacles moving, new obstacles appearing, and environmental conditions fluctuating. This dynamic nature of the environment adds an extra layer of complexity to path planning, as the robot must be able to adapt its path in real-time to avoid collisions and navigate around new obstacles. Addressing dynamic environments often requires the use of sensor technologies, such as lidar, radar, and cameras, to provide real-time data about the robot's surroundings, as well as advanced algorithms that can quickly process this data and generate new paths as needed.Furthermore, path planning in robot navigation also needs to consider real-time constraints, such as the need to make quick decisions to avoid collisions or respond to unexpected changes in the environment. In many cases, robots are required to operate inreal-time, meaning that they must be able to quickly generate and execute new paths as the situation demands. This requires the use of algorithms and technologies that can efficiently compute new paths in a matter of milliseconds, as well as hardware and software systems that can quickly translate these paths into physical movements. Addressing real-time constraints is crucial for ensuring the safety and efficiency of robot navigation in dynamic environments.In addressing these challenges, there are several approaches and technologies that are commonly used in path planning for robot navigation. One approach is the use of grid-based algorithms, which divide the robot's environment into a grid of cells and use algorithms such as A* or Dijkstra's to find the optimal path from the robot's current position to its destination. Grid-based algorithms are relatively simple and efficient, making them well-suited for static environments with known obstacles. However, they may struggle to adapt to dynamic environments and real-time constraints.Another approach is the use of sampling-based algorithms, such as Rapidly-exploring Random Trees (RRT) or Probabilistic Roadmaps (PRM), which randomly sample the robot's configuration space to generate a network of feasible paths. Sampling-based algorithms are well-suited for dynamic environments, as they can quickly adapt to changes and generate new paths as needed. However, they may struggle to find the most optimal path in complex environments with narrow passages or tight constraints.Additionally, machine learning and artificial intelligence are increasingly being used in path planning for robot navigation. These technologies can enable robots to learn from their experiences and adapt their path planning strategies based on past data. For example, reinforcement learning algorithms can enable robots to learn from trial and error, gradually improving their path planning capabilities over time. Machine learning and artificial intelligence have the potential to significantly enhance the adaptability and efficiency of path planning for robot navigation, particularly in complex and dynamic environments.In conclusion, path planning is a critical aspect of robot navigation that involves balancing between optimality, feasibility, and real-time constraints. Addressing thesechallenges requires the use of advanced algorithms, sensor technologies, and real-time computing capabilities. While there are several approaches and technologies that can be used for path planning, each has its own strengths and limitations. As robotics continues to advance, it is likely that new approaches and technologies will emerge to further enhance the capabilities of path planning for robot navigation. Ultimately, the goal is to enable robots to navigate safely and efficiently in a wide range of environments, from warehouses to city streets, ultimately improving our lives and the way we interact with technology.。
Real-time node.js Instrumentation Visualzation and Debugging Bryan Cantrill
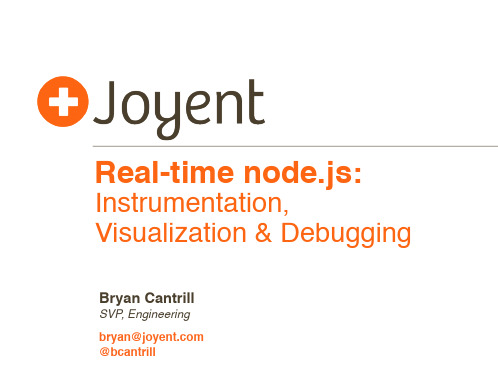
Instrumenting for latency
• • • • •
Instrumenting for latency requires modifying the system twice: as an operation starts and as it finishes During an operation, the system must track — on a peroperation basis — the start time of the operation Upon operation completion, the resulting stored data cannot be a scalar — the distribution is essential when understanding latency Instrumentation must be systemic; must be able to reach to the sources of latency deep within the system These constraints eliminate static instrumentation; we need a better way to instrument the system
One must deal not only with the traditional challenge of scalability, but also the challenge of a real-time system!
Building DIRTy apps
• • • • •
Embedded real-time systems are sufficiently controlled that latency bubbles can be architected away Web-facing systems are far too sloppy to expect this! Focus must shift from preventing latency bubbles to preventing latency bubbles from cascading Operations that can induce latency (network, I/O, etc.) must not be able to take the system out with them! Implies purely asynchronous and evented architectures, which are notoriously difficult to implement...
The Self Triggered Task Model for Real-Time Control Systems
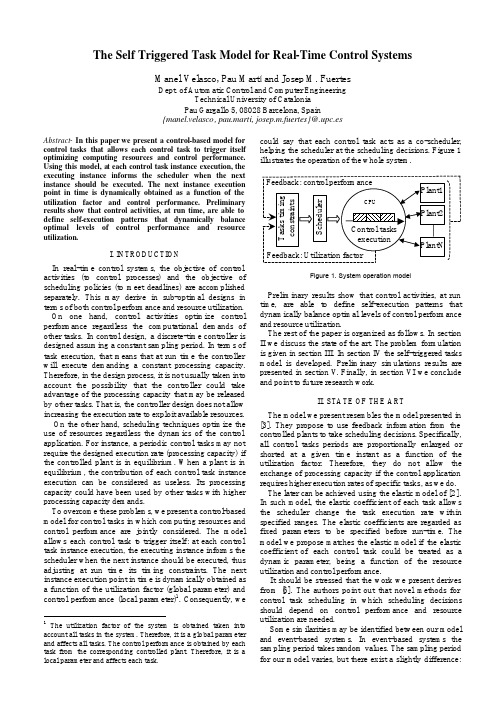
The Self Triggered Task Model for Real-Time Control Systems
Manel Velasco, Pau Martí and Josep M. Fuertes
Dept. of Automatic Control and Computer Engineering Technical University of Catalonia Pau Gargallo 5, 08028 Barcelona, Spain {manel.velasco , pau.marti, josep.m.fuertes}@.upc.es
verilog realtime用法
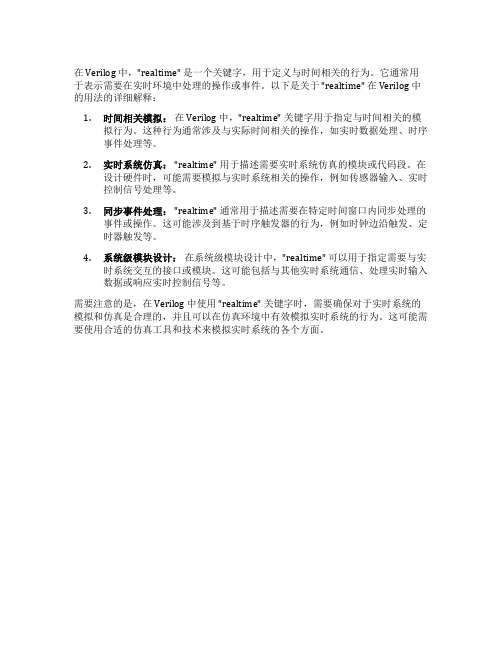
在 Verilog 中,"realtime" 是一个关键字,用于定义与时间相关的行为。
它通常用于表示需要在实时环境中处理的操作或事件。
以下是关于 "realtime" 在 Verilog 中的用法的详细解释:
1.时间相关模拟:在 Verilog 中,"realtime" 关键字用于指定与时间相关的模
拟行为。
这种行为通常涉及与实际时间相关的操作,如实时数据处理、时序事件处理等。
2.实时系统仿真: "realtime" 用于描述需要实时系统仿真的模块或代码段。
在
设计硬件时,可能需要模拟与实时系统相关的操作,例如传感器输入、实时控制信号处理等。
3.同步事件处理: "realtime" 通常用于描述需要在特定时间窗口内同步处理的
事件或操作。
这可能涉及到基于时序触发器的行为,例如时钟边沿触发、定时器触发等。
4.系统级模块设计:在系统级模块设计中,"realtime" 可以用于指定需要与实
时系统交互的接口或模块。
这可能包括与其他实时系统通信、处理实时输入数据或响应实时控制信号等。
需要注意的是,在 Verilog 中使用 "realtime" 关键字时,需要确保对于实时系统的模拟和仿真是合理的,并且可以在仿真环境中有效模拟实时系统的行为。
这可能需要使用合适的仿真工具和技术来模拟实时系统的各个方面。
TOC(约束理论)模板

TOC(约束理论)模板Title: Constraints Theory of Choice (TOC)Introduction:- Briefly introduce the Constraints Theory of Choice (TOC) as a framework for understanding decision-making processes.- Highlight its significance in various fields, including psychology, economics, and management.I. Overview of Constraints Theory:A. Definition and key concepts:1. Explain the concept of "constraints" and their role in decision-making.2. Introduce the concept of "real constraints" (e.g., time, resources) and "perceived constraints" (e.g., beliefs, attitudes).3. Discuss the importance of understanding both types of constraints for effective decision-making.B. Components of decision-making under Constraints Theory:1. Identification of objectives or desired outcomes.2. Assessment of alternative options.3. Evaluation of constraints impacting the decision-making process.4. Selection of the optimal choice given the constraints.II. Types of Constraints:A. Real constraints:1. Time constraints: Analyze how limited time influences decision-making.2. Resource constraints: Explore the impact of limited resources on choices.3. Physical constraints: Discuss how physical limitations affect decision-making.B. Perceived constraints:1. Beliefs and attitudes: Examine how personal beliefs and attitudes shape decision-making.2. Social norms and expectations: Discuss the influence of societal norms on choices.3. Emotional constraints: Highlight how emotions canimpede decision-making.III. Application of Constraints Theory:A. Psychology:1. Cognitive constraints and decision-making biases.2. Behavioral economics and constraints as determinants of choices.3. Consumer decision-making under real and perceived constraints.B. Economics:1. Game theory and constraints in strategic decision-making.2. Resource allocation and constraints in economic systems.3. Economic modeling incorporating constraints.C. Management and Leadership:1. Organizational decision-making under resource and time constraints.2. Constraints and innovation within organizations.3. Leadership decision-making and managing constraints.IV. Criticisms and Future Directions:A. Criticisms of the Constraints Theory of Choice:1. Lack of comprehensive empirical evidence.2. Difficulty in quantifying perceived constraints.B. Future directions for TOC research:1. Further empirical studies to validate the theory.2. Development of practical decision-making tools based on TOC.Conclusion:- Summarize the main points discussed in the essay regarding the Constraints Theory of Choice (TOC).- Emphasize the importance of considering both real and perceived constraints for effective decision-making.- State the potential implications of the TOC in various fields and its relevance in understanding human decision-making processes.。
实时操作系统

Real-Time Systems,28,237±253,2004#2004Kluwer Academic Publishers.Manufactured in The Netherlands. Real-Time Operating SystemsJOHN A.STANKOVICUniversity of VirginiaR.RAJKUMARCarnegie Mellon University1.IntroductionReal-time operating systems(RTOSs)provide basic support for scheduling,resource management,synchronization,communication,precise timing,and I/O.RTOSs have evolved from single-use specialized systems to a wide variety of more general-purpose operating systems(such as real-time variants of L inux).We have also seen an evolution from RTOSs which are completely predictable and support safety-critical applications to those which support soft real-time applications.Such support includes the concept of quality of service(QoS)for open real-time systems,often applied to multimedia applications as well as large,complex distributed real-time systems.Researchers in real-time operating system have developed new ideas and paradigms that enhance traditional operating systems to be more ef®cient and predictable.Some of these ideas are now found in traditional operating systems and many other ideas are found in the wide variety of RTOS on the market today.The RTOS market includes many proprietary kernels, composition-based kernels,and real-time versions of popular OSs such as Linux and Windows-NT.Many industry standards have been in¯uenced by RTOS research including POSIX real-time extensions,Real-Time Speci®cation for Java,OSEK(automotive RTOS standard),Ada83and Ada95.This paper provides an overview of the architectures, principles,paradigms,and new ideas developed in RTOS research over the past20years. The paper concentrates on research done within the context of complete RTOSs.Note that much more research on RTOSs has been accomplished and published as speci®c aspects on RTOS.For example,real-time synchronization and memory management research has many exciting results.Also,many ideas found in the companion paper on real-time scheduling can be found in various RTOSs as well.2.RTOS Taxonomy and ArchitecturesReal-time operating systems emphasize predictability,ef®ciency,and include features to support timing constraints.Several general categories of real-time operating systems exist:small,proprietary kernels(commercially available as well as homegrown kernels), real-time extensions to commercial timesharing operating systems such as Unix and238STANKOVIC AND RAJKUMAR Linux,component-based kernels,QoS-based kernels,and(largely)University-based research kernels.2.1.Small,Fast,Proprietary KernelsThe small,fast,proprietary kernels come in two varieties:homegrown1and commercial offerings.2Both varieties are often used for small embedded systems when very fast and highly predictable execution must be guaranteed.The homegrown kernels are usually highly specialized to the application.The cost of uniquely developing and maintaining a homegrown kernel,as well as the increasing quality of the commercial offerings is signi®cantly reducing the practice of generating homegrown kernels.In addition, component-based OSs(see Section2.3)are also reducing the need for homegrown kernels.For both varieties of proprietary kernels,to achieve speed and predictability,the kernels are stripped down and optimized versions of timesharing operating systems.To reduce the run-time overheads incurred by the kernel and to make the system fast,the kernel:*has a fast context switch,*has a small size(with its associated minimal functionality),*responds to external interrupts quickly(sometimes with a guaranteed maximum latency to post an event but,generally,no guarantee is given as to when processing of the event will be completed;this later guarantee can sometimes be computed if priorities are assigned correctly),*minimizes intervals during which interrupts are disabled,*provides®xed or variable sized partitions for memory management(i.e.,no virtual memory)as well as the ability to lock code and data in memory,*provides special sequential(often memory-based)®les that can accumulate data at a fast rate.To deal with timing requirements,the kernel*supports multi-tasking,*provides a priority-based preemptive scheduling mechanism,*provides bounded execution time for most primitives,*maintains a high-resolution real-time clock,REAL-TIME OPERATING SYSTEMS239 *provides for special alarms and timeouts,*supports real-time queuing disciplines such as earliest deadline®rst and primitives for jamming a message into the front of a queue,*provides primitives to delay processing by a®xed amount of time and to suspend/ resume execution.In general,the kernels also perform multi-tasking and inter-task communication and synchronization via standard primitives such as mailboxes(message queues),events, signals,mutexes,and semaphores.While all these latter features are designed to be fast, ``fast''is a relative term and not suf®cient when dealing with real-time constraints. Nevertheless,many real-time system designers use these features as a basis upon which to build real-time systems.This has been effective in small embedded applications such as instrumentation,communication front-ends,intelligent peripherals and many areas of process control.Since these applications are simple,it is relatively easy to show that all timing constraints are met.Consequently,the kernels provide exactly the minimal functionality that is needed.However,as applications become more complex,it becomes more and more dif®cult to craft a solution based on priority-driven scheduling where all timing,computation time,resource,precedence,and value requirements are mapped to a single priority for each task.In these situations,demonstrating predictability can be rather dif®cult.2.2.Real-Time Extensions to Commercial Operating SystemsA second approach to real-time operating systems is the extension of commercial products,for example,extending Unix to RT-Unix(Furht et al.,1991),Linux to RT-Linux(FSLLabs;Niehaus,KURT;RedIce Linux),or POSIX to RT-POSIX,or MACH to RT-MACH(Tokuda et al.,1990),or CHORUS to a real-time version(CHORUS system). The real-time version of commercial operating systems are generally slower and less predictable than the proprietary kernels,but have greater functionality and better software development environmentsÐvery important considerations in many large or complex applications.Another signi®cant advantage is that they are based on a set of familiar interfaces(standards)that facilitate portability.For Unix,since many variations of Unix have evolved,an IEEE standardization effort,called POSIX,has de®ned a common set of user-level interfaces for operating systems.The effort has focussed on eleven important real-time related functions:timers,priority scheduling,shared memory,real-time®les, semaphores,interprocess communication,asynchronous event noti®cation,process memory locking,asynchronous I/O,synchronous I/O,and threads.Various problems exist when attempting to convert a non real-time operating system to a real-time version.These problems can exist both at the system interface as well as in the implementation.For example,in Unix,interface problems exist in process scheduling due to the nice and setpriority primitives and its round-robin scheduling policy.In addition, the timer facilities are too coarse,memory management(of some versions)contains no240STANKOVIC AND RAJKUMAR method for locking pages into memory,and interprocess communication facilities do not support fast and predictable communication often resulting in different forms of priority inversion(Sha et al.,1990).The implementation problems include intolerable overhead, excessive latency in responding to interrupts,partly but very importantly,due to the non-preemptability of the kernel,and internal FIFO queues.These and other problems can and have been solved to result in a real-time operating system that is used for both real-time and non real-time processing.However,because the underlying paradigm of timesharing systems still exists,application developers must be careful not to use certain non real-time features that might insidiously impact the real-time tasks.Real-time capabilities can be added to operating systems in multiple ways.It is illustrative to study how many real-time versions of Linux have been created and commercialized in recent years.These versions can be grouped into the following categories.*Compliant kernels:In this approach,an existing real-time operating system is modi®ed such that L inux binaries can be run without any modi®cation.Essentially, the functionality and semantics of Linux system calls need to be appropriately emulated under the native operating system.For example,L ynxOS from L ynuxWorks adopts this approach.*Dual kernels:In this approach,a hard but thin real-time kernel sits below the native operating system(such as Linux or FreeBSD),and traps all accesses to and interrupts from the underlying hardware.The thin kernel schedules several hard real-time tasks co-located with it,and runs the native OS as its lowest priority task.As a result,native applications can be run without change,while hard real-time tasks can get excellent performance and predictability.A means of(non-real-time)communication is also provided between the thin real-time kernel and the native non-real-time kernel for data exchange purposes.The downside of this approach is that there is no memory protection between the real-time tasks and the native/thin kernels.As a result,the failure of any real-time task can lead to a complete system crash.The thin real-time kernel also needs to have its own set of device drivers for real-time functionality.RT-Linux(FSLLabs)is an example of this approach.*Core kernel modi®cations:In this approach,changes are made to the core of a non-real-time kernel in order to make it predictable and deterministic enough so as to behave as a real-time ing®xed-priority scheduling with a O(1)scheduler, employing high-resolution timers,making the kernel preemptive(so that a lower priority process in the kernel space due to an ongoing system call can be preempted by a higher priority process that becomes eligible to run),support for priority inheritance protocols to minimize priority inversion,making interrupt handlers schedulable using kernel threads,the use of periodic processes,replacing FIFO queues with priority queues and optimizing long paths through the kernel are typical means of accomplishing this goal.TimeSys L inux(based on CMU's L inux/RK (Oikawa and Rajkumar,1999)discussed in Section2.5.5)and to a smaller extent MontaVista Linux fall under this category.REAL-TIME OPERATING SYSTEMS241 *The Resource kernel approach:In this approach,the kernel is extended to provide support for resource reservations in addition to the traditional®xed-priority preemptive scheduling approach.The latter approach can run into problems when a relatively high-priority process overruns its expected execution time or even goes into an in®nite loop.Resource kernels support and enforce resource reservation,such that no misbehaving task can directly impact the timing behavior of another task.CMU's Linux/RK and its commercial cousin,TimeSys Linux,and fall into this category. ponent-BasedKernelsA number of systems such as OS-Kit(Ford et al.,1997),Coyote(Bhatti et al.,1999), PURE(Beuche et al.,1999),2K(Kon et al.,1998),MMLite(Helander and Forin,1998), and Pebble(Gabber et al.,1999)have a common intent to deal with operating system construction through composition.They de®ne OS components that can be selectively included to compose an RTOS that can be tailored to the application(s)at hand.OS-Kit provides a set of operating system components that can be combined to con®gure an operating system.However,it does not supply any rules to help build an operating system.Coyote is focussed on communication protocols,and its ability for re-con®guration might be adopted for operating system and embedded application areas. PURE is explicitly concerned with providing operating system components for con®guration and composition of operating systems for embedded applications.PURE uses an object-oriented methodology to provide different components for con®guration and customization of operating systems for embedded applications.2K emphasizes adaptability issues to allow applications to be as customizable as possible.2K is also concerned with component-based software for small mobile devices,or personal digital assistants(PDAs).To explore the concepts of a component-based RTOS,consider two component-based RTOSs in more detail.MML ite is an object-based,modular system architecture that provides a menu of components for use at compile-time,link-time,or runtime to construct a wide range of applications.A component in MML ite consists of one or more objects.Multiple objects can reside in a single namespace.When an object needs to send a message to an object in another namespace for the®rst time,a proxy object is created in the sending object's namespace that transparently handles the marshaling of parameters.A unique aspect of MMLite is its focus on support for transparently replacing components while these components are in use(mutation).MML ite uses COM interfaces, which in turn support dynamic recon®gurability on a per-object and per-component basis. However,COM does not provide protection between the components.The base menu of the MMLite system contains components for heap management,dynamic on-demand loading of new components,machine initialization,timer and interrupt drivers,scheduler, threads and synchronization,namespaces,®le system,network,and virtual memory. These components are typically very small(500±3000bytes on the386architecture), although the network component is much larger(84,832bytes on386).The resulting MMLite system can be quite small:the base system is26Kbytes on386,and20Kbytes242STANKOVIC AND RAJKUMAR on the ARM architecture.It is not clear to what extent MML ite provides users with the ability to easily select components that the MMLite developers write,and to what extent users themselves de®ne and utilize their own new components.Although there has been an apparent emphasis on developing minimal-sized components(in number of bytes), analysis tools regarding the runtime performance of components due to namespace resolution and the creation and loading of proxy objects is lacking.Pebble is a new operating system designed to be an ef®cient application-speci®c operating system and to support component-based applications.It also supports complex embedded applications.As an operating system,it adopts a microkernel architecture with a minimal privileged-mode nucleus that is only responsible for switching between protection domains.The OS functionality is provided by user-level components(servers), which can be replaced,augmented,or layered.The programming model is client/server; client components(applications)request services from system components(servers). Examples of system components are the interrupt dispatcher,scheduler,portal manager, device driver,®le system,virtual memory,and so on.The Pebble kernel and its essential components(interrupt dispatcher,scheduler,portal manager,real-time clock,console driver,and idle task)need approximately560Kbytes of ponents are like processes,where each one executes in its own protection domain(PD).In Pebble,a PD includes a page table and a set of portals.Portals provide communication between PDs.For example,if there is a portal from PD1to PD2,then a thread executing in PD1can invoke a speci®c service(entry point)of PD2.Therefore, components communicate through transferring threads from one PD to another using portals.The PD concept together with the portal concept can be understood as a component infrastructure.While Pebble PDs provide the means to isolate the components,portals provide the means for components to communicate with each other.Instantiation and management of portals are performed by an operating system component,Portal Manager.For instance,the instantiation process involves the registration of a server(any system or application component)in a portal and the request of a client for that portal.In Pebble,it is possible to dynamically load and to replace system components to ful®ll applications requirements.2.4.QoS-BasedKernelsQoS research has been extensive,®rst as applied to networking then to general distributed computing.More recently,QoS has been applied to soft real-time systems.In these systems,a guarantee is given that a certain amount of resources is assigned to a task or application.In other cases,there are differentiated guarantees meaning that certain classes of tasks are guaranteed resources compared to another class of tasks.For example, tasks dealing with the control of the plant may be required to obtain twice the resources than tasks reporting the results to a command center.The resources being controlled may just be the CPU or a set of resources.Many research results exist for developing algorithms to control the guarantees.Sometimes,these algorithms are implemented as monitors on top of an RTOS.In other cases,the algorithms may be implemented asREAL-TIME OPERATING SYSTEMS243 middleware(Brandt et al.,1998).The algorithms differ in their approach and utilize many different techniques such as fair-share scheduling(Jeffay et al.,1998),proportional scheduling(Stoica et al.,1996),rate-based scheduling(Jeffay,2001),reservations,and feedback control.In this paper,we are more interested in RTOS that incorporate QoS support such as RT Mach(Tokuda et al.,1990)and Rialto(Jones et al.,1996,1997).Both of these RTOSs allow users to negotiate with the RTOS for a certain amount of resources.RT-Mach employs reservations to support QoS.RT-Mach supports multimedia applications and both real-time and non-real-time tasks.Rialto allows for multiple,independent applications to co-exist.A system-wide planner reasons about the resource allocations between applications.This is similar to the reservation and admission control type work discussed above,but here,independent applications are supported on a single platform. Rialto also has support for overload and for re-negotiation of guarantees.2.5.Research KernelsMany past and current University-based research-oriented real-time operating systems have been developed.These projects addressed many of the following research issues including:*identifying the need for new approaches which challenge the basic assumptions made by timesharing operating systems and developing those new paradigms;*developing real-time process models:*some systems use the standard process model both to program with and at execution time,*some systems use the process model to program with but translate into a different run-time model to help support predictability and on-line guarantees, *some systems use real-time threads;*developing real-time synchronization primitives such as those that support priority inheritance and priority ceiling protocols;*developing solutions that facilitate timing analysis of both the initial system and upon modi®cations(the real-time scheduling algorithms play a large role here);*strongly emphasizing predictability not only of the kernel but also providing good support for application-level predictability;*retaining signi®cant amounts of application semantics at run time;*developing support for fault tolerance;244STANKOVIC AND RAJKUMAR *investigating object-oriented approaches;*providing support for multiprocessor and distributed real-time systems including end-to-end timing constraints;*developing support for QoS;*attempting to de®ne a real-time micro-kernel;*providing support for real-time programming languages such as the Real-Time Speci®cation of Java(JSR-00001).We survey several research projects as representative of a much wider set of work in the®eld.2.5.1.MARSThe MARS kernel(Damm et al.,1989;Kopetz et al.,1989)offers support for controlling a distributed application based entirely on the passage of time(rather than asynchronous events)from the environment.Emphasis is placed on an a priori static analysis to demonstrate that all the timing requirements are met.An important feature of this system is that¯ow control on the maximum number of events that the system handles is automatic and this fact contributes to the predictability analysis.This system is based on a paradigm,that is,the time-triggered model,that is different than what is found in timesharing systems.The scheduling approach is static and table-driven.Support for distributed real-time systems includes a hardware-based clock synchronization algorithm and a TDMA-like protocol to guarantee timely message delivery.A number of extensions to the original work have added¯exibility to handle more dynamic situations.The time-triggered approach advocated in MARS has seen success in the automotive industry and in several other safety-critical application domains.2.5.2.SPRINGThe Spring kernel(Stankovic and Ramamritham,1995;Stankovic et al.,1999)contains real-time support for multiprocessors and distributed systems.A novel aspect of the kernel is the dynamic planning-based scheduling of tasks that arrive dynamically.Such tasks are subject to admission control and dynamically acquire reservations for resources. This takes tasks'time and resource constraints into account and avoids the need to a priori compute worst-case blocking times.Safety-critical tasks are dealt with through static table-driven scheduling.The kernel also embodies a re¯ective architecture (Stankovic and Ramamritham,1995)that retains a signi®cant amount of application semantics at run time.This approach provides a high degree of¯exibility along withREAL-TIME OPERATING SYSTEMS245 support for graceful degradation.These planning and application semantic features are integrated to provide direct support for achieving both application-and system-level predictability.The kernel also uses global replicated memory to achieve predictable distributed communication.The abstractions provided by the Kernel include dynamic guarantees,reservations,planning,and end-to-end timing support.Spring,like MARS, presents a new paradigm for real-time operating systems,but unlike MARS it strives for a more¯exible combination of off-line and on-line techniques.Concepts of admission control,re¯ection and reservations found in the Spring kernel have been used by many other systems.2.5.3.ARTSThe ARTS kernel(Tokuda and Merger,1989)provides a distributed real-time computing environment that works in conjunction with the static priority-driven preemptive scheduling paradigm.The kernel supports the notion of real-time objects and real-time threads.Each real-time object is time-encapsulated.This is enforced by a time fence mechanism which provides a run-time check that ensures that the slack time is greater than the worst-case execution time for an object invocation about to be performed.If it is, the operation proceeds,else it is aborted.Each real-time thread can have a value function, timing constraints,worst-case execution time,phase,and delay value associated with it. Communication(object invocation)proceeds in a request±accept±reply fashion,but does not address deadlines for messages.A real-time transport protocol has been developed. The ARTS kernel is also tied to various tools that a priori analyze the system-wide schedulability of the system.2.5.4.HARTOSThe hexagonal architecture for real-time systems(HARTS)consists of multiple sites connected by a hexagonal mesh network.Each site may be a uniprocessor or multiprocessor and contains an intelligent network processor.The intelligent network processor handles much of the low-level communication functions.An experimental operating system called HARTOS(Kandlur et al.,1992)is a distributed real-time kernel running on HARTS.On each site,HARTOS runs in conjunction with the commercial uniprocessor OS,pSOS.Hence,by itself,HARTOS is not a full operating system.Rather, HARTOS focusses on interprocess communication,thereby providing some support for distributed real-time systems.In particular,HARTOS supports message send and receive, non-queued event signals,reliable streams,and message scheduling that provides a best-effort approach in delivering a message by its deadline.Support for fault-tolerant routing, clock synchronization,and for replicated processes are also planned.246STANKOVIC AND RAJKUMAR 2.5.5.RKIn extensions to Real-Time Mach,Mercer et al.(1994)added the notion of processor reservations based on the Liu and Layland periodic task model of each task obtaining C i units of time every T i units of time.Rajkumar et al.(1998)generalized this concept to the notion of a resource kernel,which is de®ned as one which provides guaranteed,timely and enforced access for applications to system resources.In addition,scheduling policies could be changed within the OS without affecting any guarantees.Resources that could be guaranteed access to can include CPU cycles(Rajkumar et al.,1988),disc bandwidth (Molano et al.,1997;Saewong and Rajkumar,2003),network bandwidth(Ghosh and Rajkumar,2002)or memory space(Easwaran and Rajkumar,2004).Resource reservations on multiple resources could also be combined to form a resource set to which one or more applications could be bound.An application bound to a resource set essentially has access to a``virtual machine''that comprises a time-or space-multiplexed subset of the underlying physical resources.This virtualization also enabled the binding of binary images to be bound to arbitrarily sized reservations(w/o access to source).An interesting variation of the priority inversion problem occurs when processes bound to two(or more)different reservations need to share a resource(such as the X-windows server).Solutions to this problem are also based on variants of priority inheritance and led to reservation inheritance protocols(de Niz et al.,2001).Counter-intuitive as it may seem,the reservation model of guaranteeing and enforcing C i units of time every T i units of time is not just useful for periodic tasks.It can also act as a traf®c shaper to aperiodic tasks in the exact same spirit of a deferrable(Sprunt et al., 1989)or sporadic server(Strosnider et al.,1995).3.ParadigmsReal-time operating systems utilize various paradigms.Key concepts found in these paradigms include:hard and soft real-time guarantees,admission control,re¯ection, reservations,and resource kernels.Many of these key concepts work together in achieving the overarching paradigm presented by a particular kernel.3.1.HardandSoft Real-Tim e GuaranteesIn general,the smaller,more deterministic kernels provide support for hard deadline systems.Here all the inputs and system details are known,and careful design and analysis can result in meeting hard deadline requirements.In performing the analysis it is also possible to carefully account for the kernel overheads.Safety-critical hard real-time systems also typically employ comfortable margins on resource utilization(such as ensuring that total utilization on a resource does not exceed50±60%).The larger,more dynamic,more probabilistic kernels provide support for soft real-time systems.Here quality of service guarantees are de®ned and shown to be met in aREAL-TIME OPERATING SYSTEMS247 probabilistic sense.We sometimes®nd hierarchical real-time scheduling or partitioned scheduling to handle different classes of tasks.3.2.Admission ControlAdmission control is a function that decides if new work entering the system should be admitted or not.The key ingredients of admission control include a model of the state of system resources,knowledge about the incoming request,the exact algorithm to make the admission control decision,and policies for the actions to take upon admission and upon rejection.First consider hard real-time systems.Many hard real-time systems are statically scheduled and operate in highly deterministic fashion.This facilitates the timing analysis required of these systems and there is no notion of admission control.But,many hard real-time systems operate in dynamic environments where static scheduling is too costly or rigid.What is required is a solution that enables on-line careful timing analysis and dynamic scheduling.A solution provided in the Spring kernel (Stankovic and Ramamritham,1989,1995)included the synergistic combination of admission control,resource reservation,and re¯ection;so this concept already exists in the hard real-time domain.Here the model of the state of the system is a detailed timeline that identi®es the start and®nish time(based on a worst-case execution time model)for each admitted task on each resource that it requires.Signi®cant re¯ective information is known about each incoming task because they are pre-analyzed for a particular real-time system;there are no general purpose on-the-¯y tasks created.The re¯ective information known about the requested work includes the worst-case execution time,shared data required by this task,precedence constraints,importance level,which tasks communicate with this task,deadline,etc.The algorithm is a heuristic that schedules the task on the detailed time line along with all the previously admitted tasks in such a manner that if successful,all the tasks will meet their deadlines.See Zho et al.(1987)for a detailed description of the algorithm.If the task is admitted,it has been assigned a very speci®c time-slice(although it may actually execute early under certain conditions).If it is not admitted,then a separate policy is invoked to decide what action to take.Typical actions include:try a simpler version of the task if any exists,or if the deadline is far away try to schedule the task on another node,or if the deadline is close then just reject this task. These policies can be modi®ed based on the importance of the task.The low-level details of the entire guaranteed schedule are available to the application.A large amount of application semantic information is pushed into the kernel(via the compiler and a special system description language).For example,the process control block(PCB)contains,in addition to the typical information,worst case execution times,deadline information, precedence requirements,a communication graph,fault-tolerance information,etc. Work on supporting QoS for audio and video has also used admission control and reservations.In many systems,various amounts and types of re¯ective information are also used.The typical model of the system has been utilizations identi®ed independently for multiple resources such as CPU,network bandwidth,disc,and memory.The precise admission control algorithm has varied from system to system,but it is usually based on。
Embedded Systems Unit 2 - Real TimeSystem

Are all embedded systems “real-time system?
“embedded system”= “real-time system”?? Are all embedded systems “real-time system? “embedded system”= “real-time system”??
Then here comes the name real time.
Soft vs Hard Real-Time
Soft Real-Time System
◦ Compute output response as fast as possible, but no specific deadlines that must be met.(快速响应,但没有时间限制)
RTOS执行以上任务,但被专门设计来运行非常精确的时间和高度的 可靠性要求的应用程序
RTOS
RTOS frequently operate under such constraints:RTOS经常在这样的限 制下运行: 1. Time or deadline截止时间 2. Speed速度
3. Memory内存
Embedded Systems Unit 2 - Real-Time Systems
SOUTHEAST UNIVERSITY, SUZHOU, CHINA APRIL, 2014
Real-time Systems
Real-time System
What is A Real-time System? Definition of “Real-time”?
软硬件协同设计概念与思路
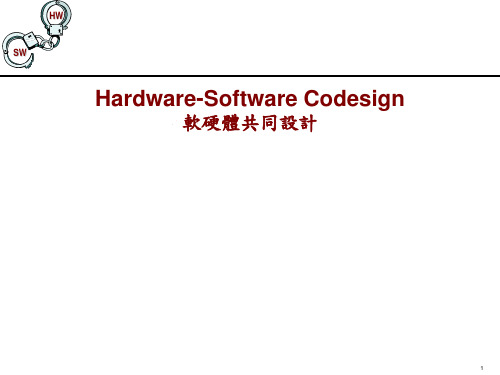
6
HW
SW
Codesign Definition and Key Concepts
Codesign
The
meeting of system-level objectives by exploiting the trade-offs between hardware and software in a system through their concurrent design
ATM Virtual Private Network Digital Camera and JPEG
5
HW
SW
Introduction to Embedded Systems and Hardware-Software Codesign
Introduction
Unified HW/SW Representations
Codesign of ISAs
Application-specific
instruction set processors (ASIPs) Compiler and hardware optimization and trade-offs
Codesign of Reconfigurable Systems
Key concepts
Concurrent:
hardware and software developed at the same time on parallel paths Integrated: interaction between hardware and software developments to produce designs that meet performance criteria and functional specifications
Components in real-time systems
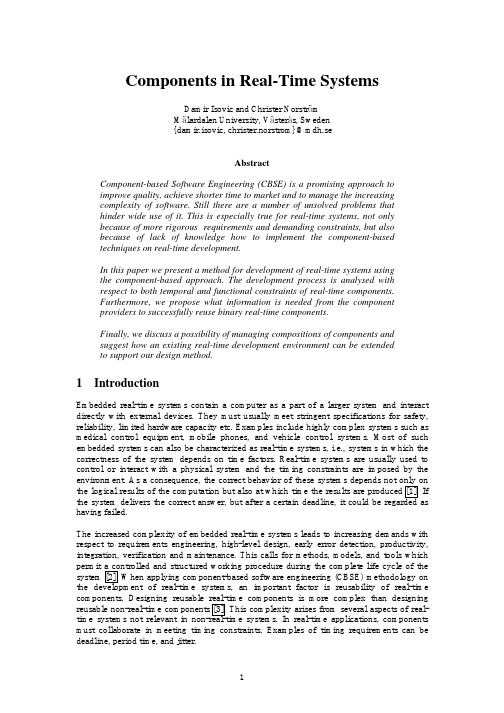
Components in Real-Time SystemsDamir Isovic and Christer NorströmMälardalen University, Västerås, Sweden{damir.isovic, christer.norstrom}@mdh.seAbstractComponent-based Software Engineering (CBSE) is a promising approach toimprove quality, achieve shorter time to market and to manage the increasingcomplexity of software. Still there are a number of unsolved problems thathinder wide use of it. This is especially true for real-time systems, not onlybecause of more rigorous requirements and demanding constraints, but alsobecause of lack of knowledge how to implement the component-basedtechniques on real-time development.In this paper we present a method for development of real-time systems usingthe component-based approach. The development process is analysed withrespect to both temporal and functional constraints of real-time components.Furthermore, we propose what information is needed from the componentproviders to successfully reuse binary real-time components.Finally, we discuss a possibility of managing compositions of components andsuggest how an existing real-time development environment can be extendedto support our design method.1 IntroductionEmbedded real-time systems contain a computer as a part of a larger system and interact directly with external devices. They must usually meet stringent specifications for safety, reliability, limited hardware capacity etc. Examples include highly complex systems such as medical control equipment, mobile phones, and vehicle control systems. Most of such embedded systems can also be characterized as real-time systems, i.e., systems in which the correctness of the system depends on time factors. Real-time systems are usually used to control or interact with a physical system and the timing constraints are imposed by the environment. As a consequence, the correct behavior of these systems depends not only on the logical results of the computation but also at which time the results are produced [1]. If having failed.The increased complexity of embedded real-time systems leads to increasing demands with respect to requirements engineering, high-level design, early error detection, productivity, integration, verification and maintenance. This calls for methods, models, and tools which permit a controlled and structured working procedure during the complete life cycle of the system [2]. When applying component-based software engineering (CBSE) methodology on components. Designing reusable real-time components is more complex than designing reusable non-real-time components [3]. This complexity arises from several aspects of real-must collaborate in meeting timing constraints. Examples of timing requirements can be deadline, period time, and jitter.Furthermore, in order to keep production costs down, embedded systems resources must usually be limited, but they must perform within tight deadlines. They must also often run continuously for long periods of time without maintenance.A desirable feature in all system development, including the development of real-time systems is the possibility of reusing standard components. However, using any particular operating system or database system for a real-time application is not always feasible, since many such systems are designed to maximize the average throughput of the system but do not guarantee temporal predictability. Therefore, to guarantee predictability, we must use either specific COTS developed for real-time systems or an appropriate subset of the functionality provided by the COTS. Some commonly used real-time COTS are real-time operating systems, communication protocols (solutions), and to some extent real-time databases. This type of components provides an infrastructure to the application. Other commonly used infrastructures in non-real-time systems are JavaBeans, CORBA and COM. However, they are seldom used for real-time systems, due to their excessive processing and memory requirements and unpredictable timing characteristics, which is of utmost importance in the class of application we consider. They have, however, one desirable property which is flexibility, but predictability and flexibility have often been considered as contradicting requirements, in particular from the scheduling perspective. Increased flexibility leads to lower predictability. Hence, a model for hard real-time systems cannot support flexibility to the same extent as the above mentioned infrastructures.Further, we require to reuse application specific components. Example of two application specific component models are IEC-1131 [5] which is a standard for programming industrial[4]. Both these models provide support for hierarchical decomposition, parameterization,similar to pipes and filters model, the difference is that the pipe only accommodates one data item, which means if the data has not already been processed when the new data arrives, it will be overwritten. However, both models lack the ability to specify timing attributes besides period time and priority which is not sufficient to specify timing sensitive systems.The development of standard real-time components which can be run on different HW platforms is complicated by the components having different timing characteristics on different platforms. Thus a component must be adapted and re-verified for each HW-platform to which it is ported, especially in safety-critical systems. Hence, we need to perform a timing analysis for each platform to which the system is ported. Given a system composed of a set of well-tested real-time components, we still face the composability problem. Besides guaranteeing the functional behavior of a specific component, the composition must also guarantee that the communication, synchronization and timing properties of the components and the system are retained. The composability problem with respect to timing properties, which we refer to as timing analysis, can thus be divided into (1) verifying that the timing properties of each component in the composed system still hold and (2) schedulability analysis (i.e. system-wide temporal attributes such as end-to-end deadlines can be fulfilled). Timing analysis is performed at two levels, the task level and the system level. At the task level the worst case execution time (WCET) for each task is either analyzed or estimated. If the execution time is measured, we can never be sure that we have determined the worst case. On the other hand if we use analysis, we must derive a safe value for the execution time. The estimated execution time must be greater than or equal to the real worst case and in the theory provided, the estimate can be excessive. The challenge here is thus to derive a value as close as possible to the real worst case execution time. Puschner gives a good introduction to this problem in the seminal paper [7]. At system level we analyze to determine if the systemexample, analysis for priority-based systems and pre-run-time scheduling techniques [8][9]. Both kinds of analysis have been proven to be useful in industrial applications [10][11]. When designing a system, we can assign time budgets to the tasks which are not implemented by intelligent guesses based on experience. By doing this we gain two positive effects. Firstly, the system level timing analysis can be performed before implementation, thus providing a tool for estimating the performance of the system. Secondly, the time budgets can be used as an implementation requirement. By applying this approach we make the design process less ad hoc with respect to real-time performance. In traditional system design, timing problems are first recognized when the complete system or subsystem has been implemented. If a timing problem is then detected, ad hoc optimization will be begun, this most surely making the system more difficult to maintain.The paper is organized as following: In Section 2 we present a method for system development using real-time components which support early analysis of the timing behavior as well as the synchronization and communication between components. The method enables high-level analysis on the architectural design level. This analysis is important to avoid costly re-design late in the development due to the detection in the integration test phase that the system as developed does not fulfill the timing requirements. The presented method is an extension of [10], and it is a standard top-down development process to which timing and other real-time specific constraints have been added and precisely defined at design time. The idea is to implement the same principles, but also taking into consideration features of existing components which might be used in the system. This means that the system is designed not only in accordance with the system requirements, but also with respect to existing components. This concept assumes that a library of well-defined real-time components is available. The development process requires a system specification, obtained by analyzing the customer's requirements.Furthermore, in Section 3, we propose a method for composing components and how the resulting compositions could be handled when designing real-time systems. In Section 4 we describe how an existing real-time development environment can be extended to support our design method. Finally, in Section 5, we provide guidelines about what one should be aware of when reusing and online updating real-time components.2 Designing component based real-time systemsIn this section we present a method for system development using real-time components. This method is an extension of [10], which is also in use in developing real-time systems within a to which timing and other real-time specific constraints have been added and precisely defined (or more correctly, have been predicted) at design time. The idea is to implement the same principles, but also taking into consideration features of existing components which might be used in the system. This means that the system is designed not only in accordance with the system requirements, but also with respect to existing components. This concept assumes that a library of well-defined real-time components is available. The development process requires a system specification, obtained by analyzing the customer's requirements. We assume that the specification is consistent and correct, in order to simplify the presentation of the method.The development process with real-time components is divided into several stages, as depicted in Figure 2-1. Development starts with the system specification, which is the input to components, the designer browses through the component-library and designs the system, making selections from the possible component candidates.Add newcomponentsto the libraryFigure 2-1: Design model for real-time componentsThe detailed design will show which components are suitable for integration. To select components, both real- and non real-time aspects must be considered. The scheduling and interface check will show if the selected components are appropriate for the system, if adaptation of components is required, or if new components must be developed. The process of component selection and scheduling may need to be repeated several times to refine the design and determine the most appropriate components. When a new component must be developed, it should be, (when developed and tested) entered into the component library. When the system finally meets the specified requirements, the timing behavior of the different components must be tested on the target platform to verify that they meet the timing constraints defined in the design phase. A detailed description of these steps is given below.2.1 Top-level designThe first stage of the development process involves de-composition of the system into manageable components. We need to determine the interfaces between them and to specify the functionality and safety issues associated with each component. Parallel with the decomposition, we browse the component library to identify a set of candidate components,(i.e., components which might be useful in our design).2.2 Detailed designAt this stage a detailed component design is performed, by selecting components to be used in each component from the candidate set. In a perfect world, we could design our system by only using the library components. In a more realistic scenario we must identify missingcomponents that we need according to our design but which are not available in the component library. Once we have identified all the components to be used, we can start by assigning attributes to them, such as time-budgets, periods, release times, precedence constraints, deadlines and mutual exclusion etc.A standard way of performing the detailed design is to use the WCET specified for every task which specifies the upper limit of the time needed to execute a task. Instead of relying on WCET values for components at this stage, a time budget is assigned to each component. A component is required to complete its execution within its time budget. This approach has also been adopted in [14], and shown to be useful in practice. Experienced engineers are often 2.3 SchedulingAt this point we need to check if the system's temporal requirements can be fulfilled, assuming time budgets assigned in the detailed design stage. In other words, we need to make a schedulability analysis of the system based on temporal requirements of each component. A scheduler which can handle the relevant timing attributes has been presented in [14], used.The scheduler in [14] takes a set of components with assigned timing attributes, and creates a static schedule. If scheduling fails, changes are necessary. It may be sufficient to revise the detailed design by reengineering the temporal requirements or by simply replacing components with others from the candidate set. An alternative is to return to top-level design and either select others from the library or specify new components.During the scheduling we must check that the system is properly integrated; component interfaces are to be checked to ensure that input ports are connected and that their types match. Further, if the specified system passes the test, besides the schedules, the infrastructure for communication between components will be generated.2.4 WCET verificationEven if the component supplier provides a specification of the WCET, it must be verified on the target platform. This is absolutely necessary when the system environment is not as in the component specification. We can verify the WCET by running test cases developed by the component designer and measuring the execution time. The longest time is accepted as the component WCET. Obtaining the WCET for a component is a quite complicated process, especially if the source code is not available for the performance of the analysis. For this reason, correct information about the WCET from the component supplier is essential.2.5 Implementation of new componentsNew components; those not already in the library must be implemented. A standard development process for the development of software components is used. It may happen that some of the new components fail to meet their assigned time budgets. The designer can either add these to the library for possible reuse in other projects or redesign them. In order to proceed, the target platform must be available at this stage. Once a component is implemented and verified we must determine its WCET on our target platform and verify the WCET of library components, if this has not been done before.2.6 System build and testFinally, we build the system using old and new components. We must now verify the functional and temporal properties of the system obtained. If the verification test fails, we must return to the appropriate stage of the development process and correct the error.2.7 Component libraryThe component library is the most central part of any CBSE system, since it contains binaries of components and their descriptions. When selecting components we examine the attributes available in the library. A component library containing real-time components should provide the following in addition to component identification, functional description, interface, component binary and test cases:• Memory requirements - Important information when designing memory restricted systems, and when performing trade-off analysis.• WCET test cases - Test cases which indicate the WCET of the components WCET for a particular processor family. Information about the WCET for previously used targets should be stored to give a sense of the components processor requirements.• Dependencies – Describing dependencies on other components.• Environment assumptions - Assumptions about the environment in which the component operates, for example the processor family.2.8 WCET test casesSince the timing behavior of components depends on both the processor and the memory organization, it is necessary to re-test the WCET for each target different from that specified. The process of finding the WCET can be a difficult and tedious process, especially if complete information or the source code is not available. Giving the WCET as a number does not provide sufficient information. What is more interesting in the test cases is the execution time behavior shown as a function of input parameters as shown in Figure 2-2. The execution time shows different values for the different input sub-domains.Execution timeInputFigure 2-2: An execution time graphProducing such a graph can also be a difficult and time-consuming process. In many cases, however, the component developer can derive WCET test cases by combining source code analysis with the test execution. For example, the developer can find that the execution time is independent of input parameters within an input range (this is possible for many “simple" processors used in embedded systems but not for others).The exact values of the execution time are not as important as the maximum value within input intervals, as depicted in Figure 2-3. When a component is instantiated, the WCET test how the component is instantiated.Execution timeInputFigure 2-3: Maximum execution time per sub-domain3 Composition of componentsAs mentioned earlier a component consists of one or more tasks. Several components can be composed into a more complex one. This is achieved by defining an interface for the new component and connecting the input and output ports of its building blocks, as shown in Figure 3-1.This new kind of component is also stored in the component library, in much the same way as the other components. However, two aspects are different: the timing information and the component binary. The WCET of a composed component cannot be computed since its parts may be executing with different periods. Instead we propose that end-to-end deadlines should be specified for the input to and output from the component. End-to-end deadlines are set such that the system requirements are fulfilled in the same way as the time budgets are set. These deadlines should be the input to a tool which can derive constraints on periods and deadlines for the sub-components. This possibility remains the subject of research and cannot be considered feasible today.Figure 3-1: Composition of componentsFurthermore, we specify virtual timing attributes (period, release time and deadline) of the composed component, which are used to compute the timing attributes of sub-components. For example, if the virtual period is set to P, then the period of a sub-component A should be f A * P and the period of B is f B * P, where f A and f B are constants for the composed component, which are stored in the component library. This enables the specification of timing attributes at the proper abstraction level. The binary of the composed component is not stored in the component library. Instead references to the sub-components are stored, to permit the retrieval of the correct set of binaries.4 Example: RT components in Rubus OSCurrently there are not so many real-time operating systems that have some concept of components. The Rubus operating system [19] is one of those. In this section we will describetogether with our development process. The scheduling theory behind this framework is explained in [14].4.1 RubusRubus is hybrid operating system, in the sense that it supports both pre-emptive static scheduling and fixed priority scheduling, also referred to as the red and blue parts of Rubus. The red part deals only with hard real-time and the blue part only with soft. Here we focus on the red part only.Each task in the red part is periodic and has a set of input and output ports, which are used for unbuffered communication with other tasks. This set also defines a task’s interface. A task provides the thread of execution for a component and the interface to other components in the system via the ports. In Figure 4-1 we can see an example of how a task/component interface could look like.Task state informationFigure 4-1: A task and its interface in the red model of RubusEach tasks has an entry function that which as arguments have input and output ports. The value of the input ports are guaranteed not to change during the execution of the current instance of the task, in order to avoid inconsistency problems. The entry function is re-invoked by the kernel periodically.The timing requirements of the component/task are shown in Figure 4-1. The timingrequirements, it is also possible to specify ordering of tasks using precedence relations, and mutual exclusion. For example the depicted task in is required to execute before the outputBrakeValues task, i.e., task BrakeLeftRight precedes task outputBrakeValues. A systemis composed of a set of components/tasks for which the input and output ports have been connected, as depicted in Figure 4-2.Figure 4-2: A composed system in the red model of RubusWhen the design of a system is finished, a pre run-time scheduler is run to check if the temporal requirements can be fulfilled. If the scheduler succeeds then it also generates a schedule for the design, which is later used by the red kernel to execute the system.4.2 Extensions for CBSELet’s see what is missing in Rubus and its supporting tools to make them more suitable for component based development. Firstly, there is currently no support for creating composite components, i.e., components that are built of other components. Secondly, some tool is needed to manage the available components and their associated source files, so that components can be fetched from a library and instantiated into new designs. Besides this there is a lack of real-time tools like: WCET analysis, allocation of tasks to nodes.Support for composition of components can easily be incorporated into Rubus, since only a front-end tool is needed that can translate component specifications to task descriptions. The front-end tool needs to perform the following for composition:1. assign a name to the new component2. specify input and output ports of the composition3. input and output ports are connected to the tasks/ components within the component,see Figure 4-3.4.Component: BrakeSystemFigure 4-3: Composition of components in Rubus5 Reuse of RT ComponentsDesign for reuse means that a component from a current project should require a minimum of modification for use in a future project. Abstraction is extremely valuable for reuse. When designing components for reuse, designers should attempt to anticipate as many future applications as possible. Reuse is more successful if designers concentrate on abstract rather than existing uses. The objective should be to minimize the difference between the component's selected and ideal degrees of abstraction. The smaller the variance from the ideal level of abstraction, the more frequently a component will be reused.There are other important factors which designers of reusable components must consider, they must not only anticipate future design contexts and future reuses. They must consider:• What users need and do not need to know about a reusable design, or how to emphasize relevant information and conceal that which is irrelevant.• What is expected from potential users, and what are their expectations about the reusable design.• That it is desirable, though difficult, to implement binary components, to allow users to instantiate only relevant parts of components. For example, if a user wants to use only some of the available ports of a component, then only the relevant parts should be instantiated.No designer can actually anticipate all future design contexts, when and in which environment the component will be reused. This means that a reusable component should depend as little as possible on its environment and be able to perform sufficient self-checking. In other words, it should be as independent as possible. Frequency of reuse and utility increase with independence. Thus independence should be another main area of concern when designing reusable components.An interesting observation about efficient reuse of real-time components, made by engineers at Siemens [15] is that, as a rule of thumb, the overhead cost of developing a reusablefifth reuse. Similar experience at ABB [16] shows that reusable components are exposed to changes more often than non-reusable parts of software at the beginning of their lives, until they reach a stable state.Designing reusable components for embedded real-time systems is even more complicated due to memory and execution time restrictions. Furthermore, real-time components must be much more carefully tested because of their safety-critical nature.These examples show that it is not easy to achieve efficient reuse, and that the development of reusable components requires a systematic approach in design planning, extensive development and support of a more complex maintenance process.5.1 Online Upgrades of ComponentsA method for online upgrades of software in safety-critical real-time systems has been presented in [17]. It can also be applied to component-based systems when replacing components.Replacing a component in a safety critical system can result in catastrophic consequences if the new component is faulty. Complete testing of new components is often not economically feasible or even possible, e.g., shutting down a process plant with high demands on availability can result in big financial losses. It is often not sufficient to simulate the behavior of the system including the new component. The real target must be used for this purpose.However, testing in the real system means that it must be shut down, and there is also a potential risk that the new component could endanger human life or vital systems.To overcome these problems it is proposed in [17] that the new component should be monitored to check that its output is within valid ranges. If it is not, then the original component will resume control of the system. It is assumed that the old component is reliable, but not as effective as the new component in some respect e.g., the new provides much improved control performance. This technology has been shown to be useful for control applications.A similar approach can be found in [18] where a component wrapper invokes a specific wrapper execution time must be taken into consideration, and such a system must support version management of components.In this development model we assume that a static schedule is used at run-time to dispatch the tasks, and since the schedule is static the flexibility is restricted. However, in some cases it is possible to perform online upgrades.Online upgrade of the system requires that the WCET of the new component is less or equal to the time-budget of the component it replaces. It is also required that it has the same interface and temporal properties, e.g., period and deadline. If this is not feasible, a new schedule must be generated and we must close down the system to upgrade it. Using the fault-tolerance method above, we can still do this safely with a short downtime.6 SummaryIn this paper we presented certain issues related to the use of component technology in the development of real-time systems. We pointed out the challenges introduced by using real-time components, such as guaranteeing the temporal behavior not only of the real-time components but also the entire composed system.When designing real-time systems with components, the design process must be changed to include timing analysis and especially to permit high-level analysis on an architectural design level. We presented a method for the development of reliable real-time systems using the component-based approach. The method emphasizes the temporal constraints which are estimated in the early design phase of the systems and are matched with the characteristics of existing real-time components. We outlined the information needed when reusing binary components, saved in a real-time component library.Furthermore, we proposed a method for composing components and how the resulting compositions could be handled when designing real-time systems. We also provided guidelines about what one should be aware of when reusing and online updating real-time components.References[1] Stankovic, J. and Ramamritham, K. Tutorial on Hard Real-Time Systems. IEEEComputer Society Press, 1998[2] D. Kalinsky and J. Ready. Distinctions between requirements specification and designof real-time systems. Conference proceedings on TRI-Ada '88 , 1988, Pages 426 – 432.[3] Douglas, B.P. Real-Time UML - Developing efficient objects for embedded systems.Addison Wesley Longman, Inc, 1998。
Specification, validation, and verification of time-critical systems
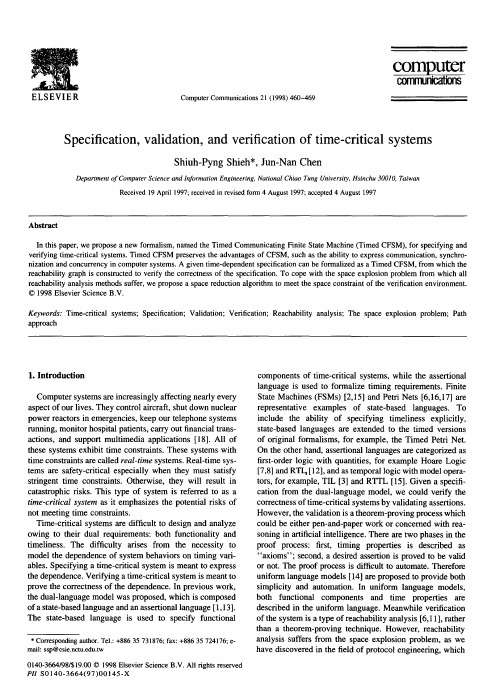
Abstract In this paper, we propose a new formalism, named the Timed Communicating Finite State Machine (Timed CFSM), for specifying and verifying time-critical systems. Timed CFSM preserves the advantages of CFSM, such as the ability to express communication, synchronization and concurrency in computer systems. A given time-dependent specification can be formalized as a Timed CFSM, from which the reachability graph is constructed to verify the correctness of the specification. To cope with the space explosion problem from which all reachability analysis methods suffer, we propose a space reduction algorithm to meet the space constraint of the verification environment. 0 1998 Elsevier Science B.V.
Departmenr of Cornpurer Science and Information Engineering, National Chiao Tung University, Hsinchu 30010, Taiwan
How to perform tasks in a critical real-time syste
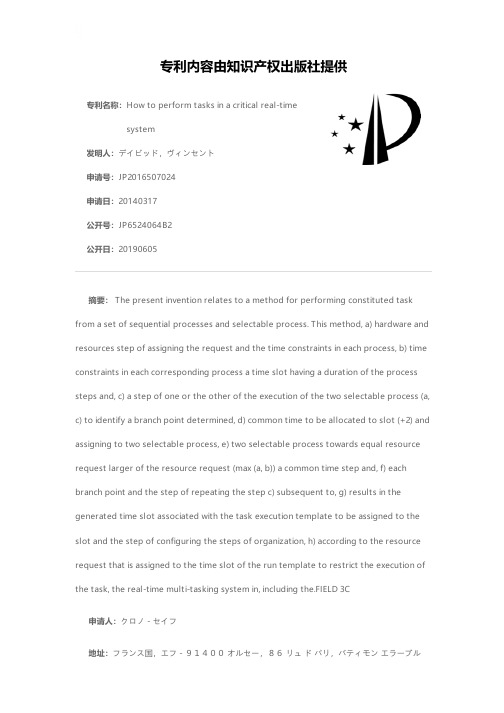
专利名称:How to perform tasks in a critical real-time system发明人:デイビッド,ヴィンセント申请号:JP2016507024申请日:20140317公开号:JP6524064B2公开日:20190605专利内容由知识产权出版社提供摘要: The present invention relates to a method for performing constituted task from a set of sequential processes and selectable process. This method, a) hardware and resources step of assigning the request and the time constraints in each process, b) time constraints in each corresponding process a time slot having a duration of the process steps and, c) a step of one or the other of the execution of the two selectable process (a, c) to identify a branch point determined, d) common time to be allocated to slot (+2) and assigning to two selectable process, e) two selectable process towards equal resource request larger of the resource request (max (a, b)) a common time step and, f) each branch point and the step of repeating the step c) subsequent to, g) results in the generated time slot associated with the task execution template to be assigned to the slot and the step of configuring the steps of organization, h) according to the resource request that is assigned to the time slot of the run template to restrict the execution of the task, the real-time multi-tasking system in, including the.FIELD 3C申请人:クロノ-セイフ地址:フランス国,エフ-91400 オルセー,86 リュ ド パリ,バティモン エラーブル国籍:FR代理人:高岡 亮一,小田 直,岩堀 明代,高橋 香元更多信息请下载全文后查看。
短视频直播利与弊英语作文
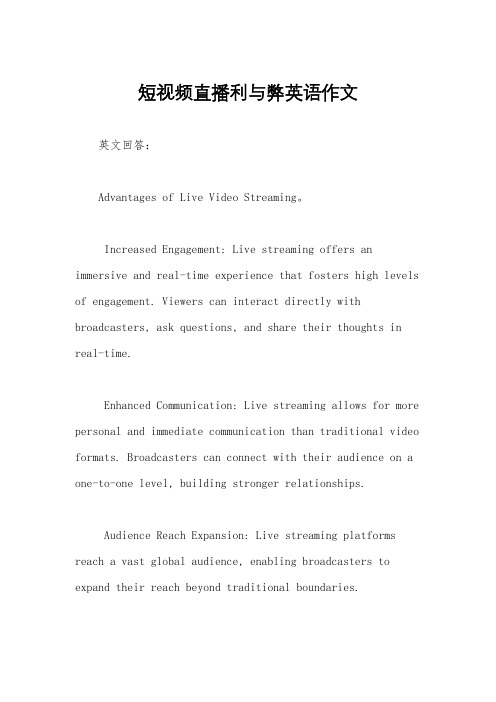
短视频直播利与弊英语作文英文回答:Advantages of Live Video Streaming。
Increased Engagement: Live streaming offers an immersive and real-time experience that fosters high levels of engagement. Viewers can interact directly with broadcasters, ask questions, and share their thoughts in real-time.Enhanced Communication: Live streaming allows for more personal and immediate communication than traditional video formats. Broadcasters can connect with their audience on a one-to-one level, building stronger relationships.Audience Reach Expansion: Live streaming platforms reach a vast global audience, enabling broadcasters to expand their reach beyond traditional boundaries.Real-Time Feedback: Viewers can provide immediate feedback during live streams, giving broadcasters valuable insights into their content's quality and effectiveness.Monetization Opportunities: Live streaming platforms offer various monetization models, such as donations, subscriptions, and sponsorships, providing opportunities for broadcasters to generate revenue.Disadvantages of Live Video Streaming。
直播的利与弊英语作文100

直播的利与弊英语作文100Benefits and Drawbacks of Livestreaming.Livestreaming, the real-time broadcasting of video and audio over the internet, has become increasingly popular in recent years, offering a range of benefits and drawbacks.Pros:Increased Engagement: Livestreams allow creators to interact directly with their audience, fostering a sense of community and building stronger relationships.Immediate Feedback: Viewers can provide immediate reactions and comments, enabling creators to adjust their content in real-time.Authenticity: Livestreams showcase real-time experiences, offering a more genuine and authentic connection with audiences.Educational Value: Livestreams can be used as a platform to share knowledge, skills, and experiences in a captivating and engaging manner.Monetization Opportunities: Livestreaming platforms often provide opportunities for creators to monetize their content through subscriptions, donations, and brand partnerships.Cons:Technical Issues: Livestreams can be susceptible to technical glitches, such as buffering, lag, and audio disruptions, which can disrupt the viewing experience.Time Constraints: Live broadcasts occur in real-time, limiting the creator's ability to edit and perfect their content before sharing it.Lack of Privacy: Livestreams are often publicly accessible, making it difficult for creators to control whohas access to their content.Potential for Cyberbullying and Harassment: Livestreams can provide a platform for cyberbullying and harassment, as viewers may make inappropriate or offensive comments in real-time.Addiction: Livestreaming can be addictive, with viewers spending excessive amounts of time watching live broadcasts.Chinese Translation:直播的利与弊。
What is Real Time and Why Do I Need It

What is Real Time and Why Do I Need It?Authored by: Steve FurrUpdated by: Thomas FletcherReal time is an often misunderstood - and misapplied - property of operating systems. In this article, I will attempt to provide a summary of some of the critical elements of realtime computing and discuss a few design considerations and benefits.What Is Real Time?We can start with a basic definition of a realtime system, as defined in the FAQ for the comp.realtime newsgroup:"A realtime system is one in which the correctness of the computations not only depends upon the logical correctness of the computation but also upon the time at which the result is produced. If the timing constraints of the system are not met, system failure is said to have occurred."Real time, then, is a property of systems where time is literally "of the essence." In a realtime system, the value of a computation depends on how timely the answer is. For example, a computation that is completed late has a diminishing value, or no value whatsoever, and a computation completed early is of no extra value. Real time is always a matter of degree, since even batch computing systems have a realtime aspect to them - nobody wants to get their payroll deposit two weeks late!Problems arise when there is competition for resources in the system and resources are shared among many activities, which is where we begin to apply the realtime property to operating systems. In implementing any realtime system, a critical step in the process will be the determination of a schedule of activities such that all activities will be completed on time.Any realtime system will comprise different types of activities - those that can be scheduled, those that cannot be scheduled, such as operating-system facilities and interrupt handlers, and non-realtime activities. If non-schedulable activities can execute in preference to schedulable activities, they will affect the ability of the system to handle time constraints.What about hard real time?Hard real time is a property of the timeliness of a computation in the system. A hard realtime constraint in the system is one for which there is no value to a computation if it is late, and the effects of a late computation may be catastrophic to the system. Simply put, a hard realtime system is one where all of the activities must be completed on time.What is soft real time?Soft real time is a property of the timeliness of a computation where the value diminishes according to its tardiness. A soft realtime system can tolerate some late answers to soft realtime computations, as long as the value hasn't diminished to zero. A soft realtime system will often carry meta requirements such as a stochastic model of acceptable frequency of late computations. Note that this is very different from conventional applications of the term, which don't account for how late a computation is completed or how frequently this may occur.Soft real time is often improperly applied to operating systems that don't satisfy the necessary conditions for guaranteeing that computations can be completed on time. Such operating systems are best described asquasi-realtime or pseudo-realtime in that they execute realtime activities in preference to others whenever necessary, but don't adequately account for non-schedulable activities in the system.Who needs real time?Traditionally, realtime operating systems have been used in "mission-critical" environments requiring hard realtime capability, where failure to perform activities in a timely manner can result in harm to persons or property.Often overlooked, however, are situations where there is a need to meet quality of service guarantees, particularly when failure to do so could result in financial penalty. This covers obvious service scenarios, such as "thirty minutes or it's free," but it also includes intangible penalties, such as lost opportunities or loss of market share.More and more, real time is being employed in consumer devices - complex systems that demand the utmost in reliability. For example, a non-realtime device aimed at presenting live video, such as MPEG movies, that depends on software for any part of the delivery of the content, may experience dropped frames at a rate that is perceived by the customer as unacceptable.In designing systems, developers need to assess whether the performance benefits warrant the use of realtime technology. A decision made early on can have unforeseen consequences when overload of the deployed system leads to pathological behavior in which most or none of the activities complete on time, if at all.Realtime technology can be applied to conventional systems in ways that have a positive impact on the user experience, either by improving the perceived response to certain events, or ensuring that important activities execute preferentially with respect to others in the system.What is a realtime OS?To the best of my knowledge, an acceptable definition of what constitutes a hard realtime operating system has never been put forward. I propose a modest definition based on realtime scheduling theory that is consistent with industry practice: A hard realtime operating system must guarantee that a feasible schedule can be executed given sufficient computational capacity if external factors are discounted. External factors, in this case, are devices that may generate interrupts, including network interfaces that generate interrupts in response to network traffic.In other words, if a system designer controls the environment of the system, the operating system itself will not be the cause of any tardy computations. We can apply this term to conventional operating systems - which typically execute tasks according to their priority - by referring to scheduling theory and deriving a minimum set of conditions that must be met. Without getting into too much detail, scheduling theory demonstrates that a schedule can be translated into static priority assignments in a way that guarantees timeliness. It does so by dividing the time available into periodic divisions and assuming a certain proportion of each division is reserved for particular realtime activities.In order to do so, the following basic requirements must be met:1. Higher-priority tasks always execute in preference to lower-priority tasks.2. Priority inversions, which may result when a higher-priority task needs a resourceallocated to a lower-priority one, are bounded.3. Non-schedulable activities, including both non-realtime activities and operating-systemactivities, don't exceed the remaining capacity in any particular division.Because of condition 3, we must discount those activities outside of the control of the operating system, yielding the external factors provision above.We can then derive the following operating system requirements (OSRs):1. The OS must support fixed-priority preemptive scheduling for tasks (both threads andprocesses, as applicable).2. The OS must provide priority inheritance or priority-ceiling emulation for synchronizationprimitives.3. The OS kernel must be preemptible.4. Interrupts must have a fixed upper bound on latency.o By extension, nested interrupt support is required.5. Operating-system services must execute at a priority determined by the client of theservice.o All services on which it is dependent must inherit that priority.o Priority inversion avoidance must be applied to all shared resources used by the service.OSR 3 and OSR 4 impose a fixed upper bound on the latency imposed on the onset of any particular realtime activity. OSR 5 ensures that operating system services themselves - which are internal factors - don't introduce non-schedulable activities into the system that could violate basic requirement 3.How does an RTOS differ from a conventional OS?The key characteristic that separates an RTOS from a conventional OS is the predictability that is inherent in all of the requirements above. A conventional OS, such as Linux, attempts to use a "fairness" policy in scheduling threads and processes to the CPU. This gives all applications in the system a chance to make progress, but doesn't establish the supremacy of realtime threads in the system or preserve their relative priorities, as is required to guarantee that they will finish on time. Likewise, all priority information is usually lost when a system service, usually performed in a kernel call, is being performed on behalf of the client thread. This results in unpredictable delays preventing an activity from completing on time.By contrast, the microkernel architecture used in the QNX RTOS is designed to deal directly with all of these requirements.The microkernel itself simply manages processes (and threads) within the system, and allows them to communicate with each other. Scheduling is always performed at the thread level, and threads are always scheduled according to their fixed priority - or, in the case of priority inversion, by the priority, as adjusted by the microkernel to compensate for priority inversions. A high-priority thread that becomes ready to run can preempt a lower-priority thread.Within this framework all device drivers and operating system services apart from basic scheduling and inter-process communication (IPC) exist as separate processes within the system. All services are accessed through a synchronous message-passing IPC mechanism that allows the receiver to inherit the priority of the client. This priority-inheritance scheme allows OSR 5 to be met by carrying the priority of the original realtime activity into all service requests and subsequent device-driver requests.There is an attendant flexibility available as well. Since OSR 1 and OSR 5 stress that device-driver requests need to operate in priority order, at the priority of the client, throughput for normal operations can be substantially reduced. Using this model, an operating service or device driver can be swapped out in favor of a realtime version that satisfies these requirements. Complex systems will generally partition such resources into realtime and non-realtime with different service and device-driver implementations for each resource.Because of the above, all activities in the system are performed at a priority determined by the thread on whose behalf they are operating.What is a soft realtime OS?A soft realtime OS must be capable of doing effectively everything that a hard realtime OS must do. In addition, a soft realtime OS must be capable of providing monitoring capabilities with accurate cost accounting on the tasks in the system. It must determine when activities have failed to complete on time or when they have exceeded their allocated CPU capacity, and trigger the appropriate response.How does all of this affect my application?If you are writing an application or system for deployment on a realtime OS, it is important to consider the effect that the RTOS characteristics have on the execution of the application, and to understand how it can be used to your benefit. For example, with an RTOS you can increase responsiveness of certain operations initiated by the user.Most applications will normally run at the default user priority within the system. This means that applications normally run in a round robin execution, competing with each other for a proportion of the CPU capacity. Without the type of realtime schedule mentioned above, you can manipulate the priorities of the processes in the system to have certain activities run preferentially to others in the system. Manipulation of the priorities is a double-edged sword. Used judiciously, it can dramatically improve response in areas that are important to the user. At the same time, it is possible to "starve" other processes in the system in a way that typically doesn't happen on a conventional desktop system.The key to ensuring that higher-priority processes and threads don't starve out other processes in the system is to be certain of the limits imposed on their execution. By pacing the execution, or by throttling it in response to load, you can limit the proportion of CPU consumed by these activities so user processes get their share of the CPU.Media players, such as audio players (MP3, .wav, etc.) and video (MPEG-2), are a good example of applications that can benefit from priority manipulation. The operation of a media player can be tied to the media rate that is required for proper playback (i.e. 44kHz audio, 30 fps video). So within this constraint, a reader thread that buffers data and a rendering or playback thread can both be designed to awaken on a programmable timer, buffer or render a single frame, and then go to sleep awaiting the next timer trigger. This provides the necessary pacing, so that the priority can be assigned above normal user activities, but below more critical system functions.By choosing appropriate priorities, playback will occur consistently at the given media rate. A well-written media player will also take into account quality of service, so that if it doesn't receive adequate CPU time, it can reduce its requirements by selectively dropping samples or follow an appropriate fall-back strategy. This will then prevent it from starving other processes as well.You may also wish to treat certain user events preferentially within the system. This works well when you increase the concurrency within an application, and when the event can always be handled in a predictable, small amount of time. The key concern here is the frequency at which these events can be generated. If they can't occur too frequently, it is safe to raise the priority of the thread responding to them. If they can occur too frequently, other activities will be starved under overload conditions.The simplest solution is to divide responsibility for events into different handling threads with different priorities and queue requests or deliver them with messages. You can tie the handler's execution to a timer, so that the execution of the thread is throttled by the timer, handling a fixed number of requests within a given interval. This stresses the importance of factoring areas of application responsibility, giving a flexibledesign with opportunities for effective use of concurrency and preferential response, all of which lead to a greater feel of "responsiveness."。
关于任务教学法的英文作文
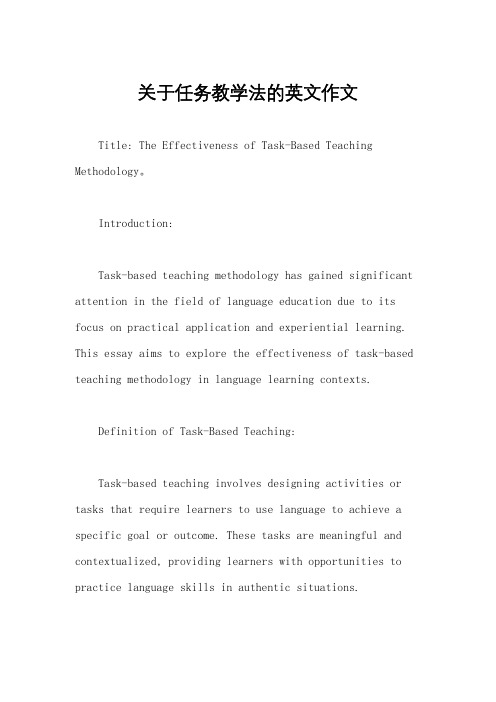
关于任务教学法的英文作文Title: The Effectiveness of Task-Based Teaching Methodology。
Introduction:Task-based teaching methodology has gained significant attention in the field of language education due to its focus on practical application and experiential learning. This essay aims to explore the effectiveness of task-based teaching methodology in language learning contexts.Definition of Task-Based Teaching:Task-based teaching involves designing activities or tasks that require learners to use language to achieve a specific goal or outcome. These tasks are meaningful and contextualized, providing learners with opportunities to practice language skills in authentic situations.Advantages of Task-Based Teaching Methodology:1. Promotes Active Learning:Task-based teaching encourages active participation and engagement among learners. By working on tasks that mimic real-life situations, students are motivated to use language creatively and effectively to accomplish the task at hand.2. Focuses on Communication:One of the key principles of task-based teaching is to prioritize communication over accuracy. Learners are encouraged to focus on conveying meaning and achieving communicative goals rather than worrying about making mistakes. This approach fosters fluency and confidence in language use.3. Integrates Language Skills:Tasks in task-based teaching typically involve theintegration of multiple language skills, such as speaking, listening, reading, and writing. This integration reflects real-world language use and helps learners develop aholistic understanding of language.4. Encourages Collaboration:Many tasks in task-based teaching involve collaborative activities where learners work together to complete a task. Collaboration not only promotes social interaction but also allows learners to learn from each other and negotiate meaning in the target language.5. Provides Meaningful Context:Tasks in task-based teaching are designed to be meaningful and relevant to learners' interests, needs, and experiences. This contextualized approach helps learners see the practical value of language learning and promotes deeper engagement with the material.Challenges and Considerations:1. Task Design:Designing effective tasks requires careful consideration of learners' proficiency levels, learning objectives, and interests. Tasks should be challenging yet achievable and should provide opportunities for meaningful language use.2. Assessment:Assessing language learning outcomes in task-based teaching can be challenging due to the focus on communication and fluency over accuracy. Assessments need to be carefully designed to evaluate both linguistic and communicative competence.3. Time Constraints:Implementing task-based teaching can be time-consuming, as designing and conducting tasks may require more time than traditional teaching methods. Teachers need to planeffectively to ensure that tasks are integrated seamlessly into the curriculum.4. Teacher Training:Effective implementation of task-based teaching requires teachers to have training and support in task design, facilitation, and assessment. Professional development opportunities can help teachers enhance their skills in implementing task-based approaches.Conclusion:Overall, task-based teaching methodology offers numerous advantages in language learning contexts, including promoting active learning, communication, integration of language skills, collaboration, and providing meaningful context. However, effective implementation requires careful task design, assessment strategies, consideration of time constraints, and ongoing teacher training and support. By embracing task-based teaching, educators can create dynamic and engaginglanguage learning experiences that better prepare learners for real-world communication.。
Real-Time Systems and Embedded Systems
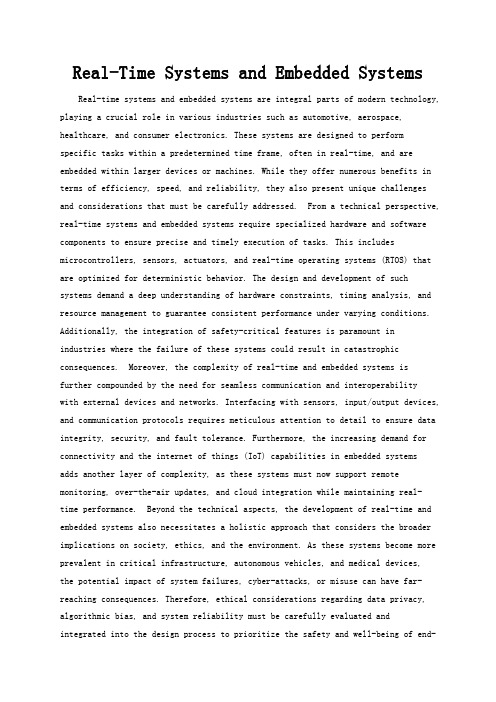
Real-Time Systems and Embedded Systems Real-time systems and embedded systems are integral parts of modern technology, playing a crucial role in various industries such as automotive, aerospace, healthcare, and consumer electronics. These systems are designed to performspecific tasks within a predetermined time frame, often in real-time, and are embedded within larger devices or machines. While they offer numerous benefits in terms of efficiency, speed, and reliability, they also present unique challenges and considerations that must be carefully addressed. From a technical perspective, real-time systems and embedded systems require specialized hardware and software components to ensure precise and timely execution of tasks. This includes microcontrollers, sensors, actuators, and real-time operating systems (RTOS) that are optimized for deterministic behavior. The design and development of such systems demand a deep understanding of hardware constraints, timing analysis, and resource management to guarantee consistent performance under varying conditions. Additionally, the integration of safety-critical features is paramount inindustries where the failure of these systems could result in catastrophic consequences. Moreover, the complexity of real-time and embedded systems isfurther compounded by the need for seamless communication and interoperabilitywith external devices and networks. Interfacing with sensors, input/output devices, and communication protocols requires meticulous attention to detail to ensure data integrity, security, and fault tolerance. Furthermore, the increasing demand for connectivity and the internet of things (IoT) capabilities in embedded systemsadds another layer of complexity, as these systems must now support remote monitoring, over-the-air updates, and cloud integration while maintaining real-time performance. Beyond the technical aspects, the development of real-time and embedded systems also necessitates a holistic approach that considers the broader implications on society, ethics, and the environment. As these systems become more prevalent in critical infrastructure, autonomous vehicles, and medical devices,the potential impact of system failures, cyber-attacks, or misuse can have far-reaching consequences. Therefore, ethical considerations regarding data privacy, algorithmic bias, and system reliability must be carefully evaluated andintegrated into the design process to prioritize the safety and well-being of end-users. Furthermore, the environmental impact of real-time and embedded systems cannot be overlooked, particularly as the proliferation of electronic devices continues to rise. The responsible use of resources, energy-efficient design, and proper disposal of electronic waste are essential considerations for sustainable development. The longevity and upgradability of embedded systems also play a significant role in reducing electronic waste and promoting a circular economy, thereby minimizing the environmental footprint of these technologies. In conclusion, the development and deployment of real-time and embedded systems require a multifaceted approach that encompasses technical, ethical, and environmental considerations. As these systems continue to evolve and permeate various aspects of our lives, it is imperative to strike a balance between innovation and responsibility to ensure that they contribute to the betterment of society. By addressing the challenges and embracing the opportunities presented by real-time and embedded systems, we can harness their full potential while mitigating potential risks and creating a sustainable future for generations to come.。
- 1、下载文档前请自行甄别文档内容的完整性,平台不提供额外的编辑、内容补充、找答案等附加服务。
- 2、"仅部分预览"的文档,不可在线预览部分如存在完整性等问题,可反馈申请退款(可完整预览的文档不适用该条件!)。
- 3、如文档侵犯您的权益,请联系客服反馈,我们会尽快为您处理(人工客服工作时间:9:00-18:30)。
†
Contents Abstract......................................................................................................1 1 2 Introduction.........................................................................................3 Un-timed and Real-Time Systems.....................................................5
References ................................................................................................28
2
1. Introduction
Should real-time be fast? No, that is a myth! In fact, real-time is just in time. For example, an avionics flight control system must spend 2.5 ms for attitude control every 50 ms period and 0.28 ms for flutter control every 4 ms period. The period restriction must be strictly abided, no faster and no slower, otherwise an airplane might crash! These just-in-time aspects constitute what are called real-time constraints. With the increased use of intelligent everyday-life systems, such as electric home appliances, office automation contrivances, and medical equipments, it has become increasingly important to assimilate knowledge on how real-time constraints can be specified, modeled, implemented, and verified. Without trying to be exhaustive, this article tries to cover most aspects of real-time constraints, such that both a novice and an expert may benefit from referring to it. Informally speaking, a real-time constraint is any condition on the timing of events, including event enabling, firing, initiation, resource usage, synchronization, and termination. A real-time constraint may be as simple as the specification of a deadline for a particular task to complete execution. A periodic real-time task may be further associated with a period constraint. For example, task A must execute once every 50 ms period with a deadline of 60 ms. Further details on the specification of real-time constraints are given in Section 3. Constraints may be classified in several ways [1]. As far as strictness of timing is concerned, constraints may be classified as hard or soft. Hard constraints must be satisfied, the failure of which results in system crashes or serious consequences. Soft constraints may be satisfied and have tolerance ranges associated, the violation of which merely degrades a system behavior, without endangering it or the environment. Hard real-time constraints are found in high-assurance systems, such as nuclear reactors, avionics, power systems, medical emergency equipments, and space navigation systems. Soft real-time constraints are found in low-assurance systems, such as telecommunication systems, network systems, electric home appliances, flexible manufacturing systems, and office automation systems. Constraints can also be classified based on the type of specification. There are two types: (1) abstract specifications in the form of an assertion language that is independent from the design or implementation language, and (2) integrated specifications that are inseparable from the implementation language andeal-Time System Design.................................................................17
4.1 4.2 4.3 Real-Time Scheduling ............................................................................... 17 Hardware System Design .......................................................................... 20 Software System Design ............................................................................ 21 4.3.1 4.3.2 4.3.3 4.3.4 Formal Software Synthesis.................................................................21 Object-Oriented Application Frameworks ........................................22 Real-Time Operating Systems ............................................................23 Hardware-Software Co-design...........................................................23
The author will be moving to the Department of Computer Science and Information Engineering, National Chung-Cheng University, Chiayi, Taiwan, starting February 2001. The work was supported by several research grants from the National Science Council, Taipei, Taiwan. 1
Real-Time Constraints
Pao-Ann Hsiung† Institute of Information Science, Academia Sinica, Taipei, Taiwan, ROC E-mail: hpa@
Abstract
Real-time constraints are restrictions on the timings of events, such that they occur on-time. A system with real-time constraints is called a real-time system. Not merely the performance of such systems, but also their feasibility depends on the satisfaction of real-time constraints. Hard constraints must be satisfied for system correctness, while the violation of soft constraints only degrades a system. Specification of real-time constraints requires either some extensions of programming languages through annotations and logic expressions, or the use of temporal logics or some formal declarative language with temporal constructs. Stringent timing restrictions complicate system design and verification. The time model can be dense or discrete, thus giving different methods for real-time system synthesis and verification. This article surveys the specification, design, and verification of real-time constraints and systems. The most important technique for guaranteeing real-time, namely scheduling, is briefly surveyed. Different system models are presented for handling real-time constraints such as Petri nets, timed automata, process algebra and object-oriented model. Design techniques for real-time hardware systems and for real-time software applications are introduced and discussed. Hardware-software codesign is also introduced. Verification techniques for real-time constraints, such as model checking, are also presented.