A nano-structured and highly ordered polypyrrole-sulfur cathode for
波导特性
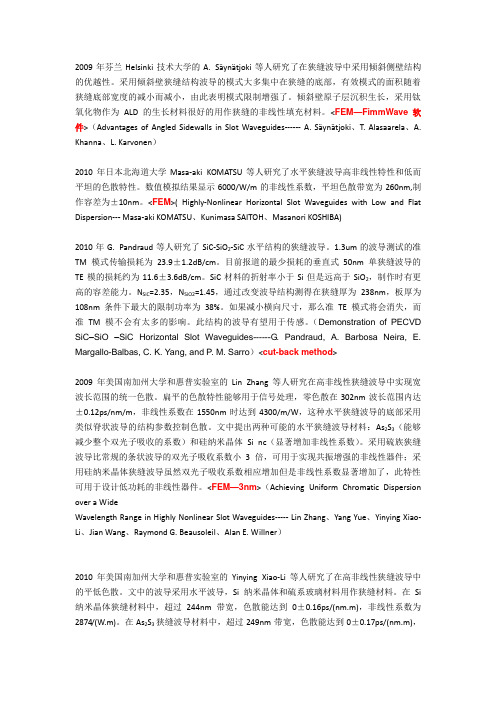
2009年芬兰Helsinki技术大学的A. Säynätjoki等人研究了在狭缝波导中采用倾斜侧壁结构的优越性。
采用倾斜壁狭缝结构波导的模式大多集中在狭缝的底部,有效模式的面积随着狭缝底部宽度的减小而减小,由此表明模式限制增强了。
倾斜壁原子层沉积生长,采用钛氧化物作为ALD的生长材料很好的用作狭缝的非线性填充材料。
<FEM—FimmWave 软件>(Advantages of Angled Sidewalls in Slot Waveguides------ A. Säynätjoki、T. Alasaarela、A. Khanna、L. Karvonen)2010年日本北海道大学Masa-aki KOMATSU等人研究了水平狭缝波导高非线性特性和低而平坦的色散特性。
数值模拟结果显示6000/W/m的非线性系数,平坦色散带宽为260nm,制作容差为±10nm。
<FEM>( Highly-Nonlinear Horizontal Slot Waveguides with Low and Flat Dispersion--- Masa-aki KOMATSU、Kunimasa SAITOH、Masanori KOSHIBA)2010年G. Pandraud等人研究了SiC-SiO2-SiC水平结构的狭缝波导。
1.3um的波导测试的准TM模式传输损耗为23.9±1.2dB/cm。
目前报道的最少损耗的垂直式50nm单狭缝波导的TE模的损耗约为11.6±3.6dB/cm。
SiC材料的折射率小于Si但是远高于SiO2,制作时有更高的容差能力。
N SiC=2.35,N SiO2=1.45,通过改变波导结构测得在狭缝厚为238nm,板厚为108nm条件下最大的限制功率为38%。
如果减小横向尺寸,那么准TE模式将会消失,而准TM模不会有太多的影响。
纳米结构材料及其技术在太阳能电池中的应用和发展现状

纳米结构材料及其技术在太阳能电池中的应用和发展现状王二垒,张秀霞,杨小聪,张绍慧(北方民族大学电信学院,宁夏银川750021)摘要:太阳能电池的发展和利用离不开太阳能电池材料和技术的发展,文中对纳米结构材料及其技术在太阳能电池和太阳能光电转化技术中的应用和发展现状做了简要综述。
介绍了多元化合物太阳电池纳米材料、染料敏化太阳电池纳米材料和有机聚合物太阳电池结构纳米材料的研究现状和技术创新,并指出其发展趋势。
关键词:太阳能电池;阳能电池材料;纳米结构材料;光电转化中图分类号:O 484.4文献标识码:A文章编号:1674-6236(2012)24-0184-04Application and development of NANO -structured materials andtechnologies for solar cellsWANG Er -lei ,ZHANG Xiu -xia ,YANG Xiao -cong ,ZHANG Shao -hui(School of Electronics and Information Engineering ,North National University ,Yinchuan 750021,China )Abstract:The development and use of solar cells can not be separated from the development of solar materials and technologies ,this paper summarized the application and development of NANO -structured material and technologies for solar cells and solar photoelectric conversion.The study status and technology innovation for multi -element compounds solar cells of NANO -structured materials ,dye -sensitized solar cells of NANO -structured materials and organic polymer solar cells of NANO -structured materials were introduced ,the development tendency were also been pointed out.Key words:solar cells ;solar cell material ;NANO -structured material ;photoelectric conversion收稿日期:2012-08-28稿件编号:201208157基金项目:国家自然科学基金资助项目(60844006);北方民族大学研究生创新项目(2012XYC040;2012XYC041);宁夏高等学校科学研究项目基金(2011JY002);北方民族大学科学研究专项任务项目基金(2011XJZKJ02);北方民族大学大学生创新项目(CJJ-CX-DX-40;CJJ-CX-DX-39)作者简介:王二垒(1985—),男,河南商水人,硕士研究生。
国内外材料领域的牛人
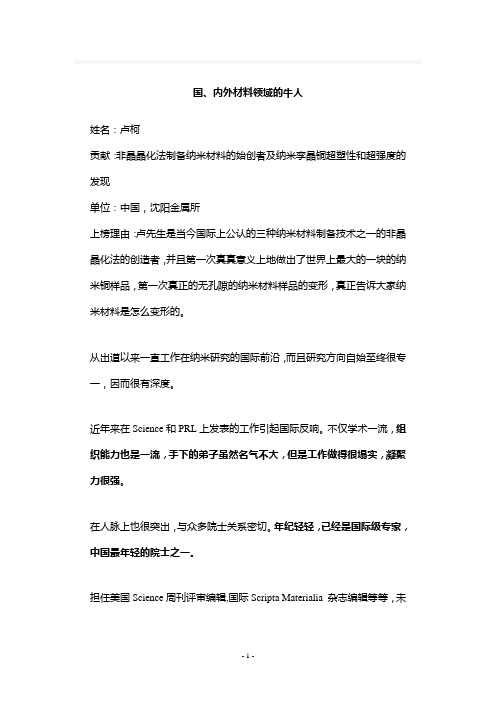
国、内外材料领域的牛人姓名:卢柯贡献:非晶晶化法制备纳米材料的始创者及纳米孪晶铜超塑性和超强度的发现单位:中国,沈阳金属所上榜理由:卢先生是当今国际上公认的三种纳米材料制备技术之一的非晶晶化法的创造者,并且第一次真真意义上地做出了世界上最大的一块的纳米铜样品,第一次真正的无孔隙的纳米材料样品的变形,真正告诉大家纳米材料是怎么变形的。
从出道以来一直工作在纳米研究的国际前沿,而且研究方向自始至终很专一,因而很有深度。
近年来在Science和PRL上发表的工作引起国际反响。
不仅学术一流,组织能力也是一流,手下的弟子虽然名气不大,但是工作做得很塌实,凝聚力很强。
在人脉上也很突出,与众多院士关系密切。
年纪轻轻,已经是国际级专家,中国最年轻的院士之一。
担任美国Science周刊评审编辑,国际Scripta Materialia 杂志编辑等等,未来的中国纳米材料第一人!代表性文章:1,K. Lu, L. Lu, S. SureshStrengthening Materials by Engineering Coherent Internal Boundaries at the NanoscaleScience,324 (2009) 349-3522,L. Lu, X. Chen, X. Huang, K. LuRevealing the Maximum Strength in Nanotwinned CopperScience, 323 (2009) 607-6103,L. LU, Y.F. SHEN, X.H. CHEN, L.H. QIAN, K. LUUltrahigh Strength and High Electrical Conductivity in CopperScience, 304 (2004) 422-4264,L. LU, M.L. SUI, K. LUSuperplastic extensibility of nanocrystalline copper at room temperature Science, 287 (2000) 1463-1466Last edited by yangruirui on 2009-5-20 at 21:54 ]姓名:王中林贡献:半导体纳米材料、纳米生物技术单位:美国,佐治亚理工学院上榜理由:王教授是国内外知名学者。
aao模板法制备纳米材料流程
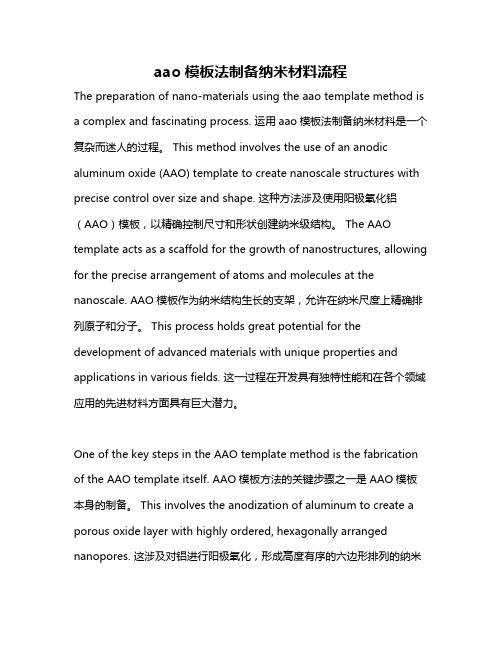
aao模板法制备纳米材料流程The preparation of nano-materials using the aao template method is a complex and fascinating process. 运用aao模板法制备纳米材料是一个复杂而迷人的过程。
This method involves the use of an anodic aluminum oxide (AAO) template to create nanoscale structures with precise control over size and shape. 这种方法涉及使用阳极氧化铝(AAO)模板,以精确控制尺寸和形状创建纳米级结构。
The AAO template acts as a scaffold for the growth of nanostructures, allowing for the precise arrangement of atoms and molecules at the nanoscale. AAO模板作为纳米结构生长的支架,允许在纳米尺度上精确排列原子和分子。
This process holds great potential for the development of advanced materials with unique properties and applications in various fields. 这一过程在开发具有独特性能和在各个领域应用的先进材料方面具有巨大潜力。
One of the key steps in the AAO template method is the fabrication of the AAO template itself. AAO模板方法的关键步骤之一是AAO模板本身的制备。
This involves the anodization of aluminum to create a porous oxide layer with highly ordered, hexagonally arranged nanopores. 这涉及对铝进行阳极氧化,形成高度有序的六边形排列的纳米孔的多孔氧化物层。
A highly ordered nanostructured carbon–sulphur cathode for lithium–sulphur batteries

A highly ordered nanostructured carbon–sulphur cathode for lithium–sulphur batteriesXiulei Ji,Kyu Tae Lee and Linda F.Nazar *The Li–S battery has been under intense scrutiny for over two decades,as it offers the possibility of high gravimetric capacities and theoretical energy densities ranging up to a factor of five beyond conventional Li-ion systems.Herein,we report the feasibility to approach such capacities by creating highly ordered interwoven composites.The conductive mesoporous carbon framework precisely constrains sulphur nanofiller growth within its channels and generates essential electrical contact to the insulating sulphur.The structure provides access to Li +ingress/egress for reactivity with the sulphur,and we speculate that the kinetic inhibition to diffusion within the framework and the sorption properties of the carbon aid in trapping the polysulphides formed during redox.Polymer modification of the carbon surface further provides a chemical gradient that retards diffusion of these large anions out of the electrode,thus facilitating more complete reaction.Reversible capacities up to 1,320mA h g −1are attained.The assembly process is simple and broadly applicable,conceptually providing new opportunities for materials scientists for tailored design that can be extended to many different electrode materials.Safe,low-cost,high-energy-density and long-lasting recharge-able batteries are in high demand to address pressing environmental needs for energy storage systems that can be coupled to renewable sources 1,2.These include wind,wave and solar energy,as well as regenerative braking from vehicular transport.With production of oil predicted to decline,and the number of vehicles and their pollution impact increasing globally,a transformation in transportation economy is inevitable given that we live in a carbon-constrained world.One of the most promising candidates for storage devices is the lithium–sulphur cell.Under intense scrutiny for well over two decades,the cell in its simplest configuration consists of sulphur as the positive electrode and lithium as the negative electrode 3,4.It differs from conventional lithium-ion cells,which operate on the basis of topotactic inter-calation reactions:reversible uptake of Li ions and electrons in a solid with minimal change to the structure.They typically use a lithium transition-metal oxide or phosphate as a positive electrode (cathode)that de/re-intercalates Li +at a high potential with respect to the carbon negative electrode (anode).As the reaction is topotac-tic at both electrodes,the charge storage capability is inherently limited to about 300mA h g −1for any prospective system,and maximum capacities observed so far are 180mA h g −1with high power characteristics having been reported 5.The lithium–sulphur cell operates quite differently.The redox couple,described by the reaction S 8+16Li ↔8Li 2S lies near 2.2V with respect to Li +/Li o ,a potential about 2/3of that exhibited by conventional positive electrodes 6.However,this is offset by the very high theoretical capacity afforded by the non-topotactic ‘assimilation’process,of 1,675mA h g −1.Thus,compared with intercalation batteries,Li–S cells have the opportunity to provide a significantly higher energy density (a product of capacity and voltage).Values can approach 2,500W h kg −1or 2,800W h l −1on a weight or volume basis respec-tively,assuming complete reaction to Li 2S (refs 7,8).Despite its considerable advantages,the Li–S cell is plagued with problems that have hindered its widespread practical realization.These arise from the fact that all components of the cell must be addressed as a whole,including the interfaces betweenUniversity of Waterloo,Department of Chemistry,Waterloo,Ontario N2L 3G1,Canada.*e-mail:lfnazar@uwaterloo.ca.them.Sulphur or sulphur-containing organic compounds are highly electrically and ionically insulating 9.To enable a reversible electrochemical reaction at high current rates,the sulphur must maintain intimate contact with an electrically conductive additive.Various carbon–sulphur composites have been used for this purpose,but they have limitations owing to the scale of the contact area.Typical reported capacities are between 300and 550mA h g −1at moderate rates 10.To make a sulphur-containing cathode ionically conductive,liquid electrolytes are used that act not only as a charge transport medium but also as ionic conductors within the sulphur-containing cathode 11.This presents difficulties of electrolyte access.Another major hurdle is capacity degradation on repeated discharge–charge of the cell.This is mainly due to the high solubility of the polysulphide anions formed as reaction intermediates in both discharge and charge processes in the polar organic solvents used in electrolytes 12.During cycling,the polysulphide anions can migrate through the separator to the Li negative electrode whereupon they are reduced to solid precipitates (Li 2S 2and/or Li 2S),causing active mass loss.In addition,the solid product that extensively precipitates on the surface of the positive electrode during discharge becomes electrochemically irreversible,which also contributes to active mass loss 13.In response to these considerable challenges,novel advances in materials design such as new electrolytes 14–17and protective films for the lithium anode have been developed 18–binations of electrolyte modification,additives and anode protection have resulted in some promising results,although rates are not given 21.Much of the difficulty still remains at the cathode,where the lack of breakthroughs has led to some cell configurations in which all of the sulphides are solubilized (so-called ‘catholyte’cells)22.In the opposite approach,that is,to contain the sulphides,some interesting cathode developments have been reported recently 23–26.However,they still fall short of the mark for practical electrochemical performance.They include,for example,the fabrication of disordered mesoporous carbon/sulphur 50:50composites in conjunction with ionic liquid electrolytes;systems that achieve high initial capacity,but suffer extensive capacity0.20.40.60.81.01.21.4x in Li x S CMK¬3+S mixtureCMK¬3/S 155 °CV o l t a g e (V ) v e r s u s L i /L i +V o l t a g e (V ) v e r s u s L i /L i +Specific capacity (mA h g ¬1)2004006008001,0001,200Specific capacity (mA h g ¬1)02004006008001,0001,2001.52.02.53.01.52.02.53.0Sab cd2 µm2 µmFigure 1|SEM images of CMK-3/sulphur,and its electrochemical characterization.a ,Mixture of CMK-3and elemental sulphur before heating.b ,CMK-3/S heated at 155◦C,showing the disappearance of the sulphur mass indicated by the red rectangle in a .c ,d ,Comparison of the galvanostatic discharge–charge profiles of the first cycles of the carbon–sulphur composites shown in a ,b ,at a current rate of 168mAg −1.The marked increase in capacity in d is due to the encapsulation effect.fading posites with sulphur embedded in conducting polymers have shown some promising results 27.However,a large polarization was observed,resulting in a very low operating voltage that reduces the energy density of cells.The loading of active mass in the S-polymer composite is also limited (less than 55wt%)owing to the low surface area of the conducting polymer.Here,we demonstrate that cathodes based on nanostructured sulphur/mesoporous carbon materials can overcome these challenges to a large degree,and exhibit stable,high,reversible capacities (up to 1,320mA h g −1)with good rate properties and cycling efficiency.Our proof-of-concept studies are based on CMK-3,the most well-known member of the mesoporous carbon family,although they are not limited to this material.Highly ordered mesoporous carbons exhibit a uniform pore diameter,very high pore volume,interconnected porous structure and can exhibit high conductivity 28,29.They,and their oxide analogues 30,31,have attracted much attention recently as nanoscale electrode materials in Li batteries 32,33,as supercapacitors and as supports for proton-exchange-membrane fuel-cell catalysts 34.CMK-3was synthesized by a nanocasting method that uses silaceous SBA-15as a hard template.The resulting replica comprises an assembly of hollow 6.5-nm-thick carbon rods separated by empty 3–4-nm-wide channel voids 35.The channel space is spanned by carbon microfibres that prevent the collapse of the nano-architecture of the two-dimensional hexagonally ordered carbon rods.We tuned the synthesis of the CMK-3to produce a short rod-like morphology,to optimize access to the mesoporous channels 36.The CMK-3/sulphur composite was prepared following a simple melt-diffusion strategy.A 3:7weight ratio mixture of CMK-3and sulphur was heated just above the melting point of sulphur,where the viscosity is lowest 37.The melt is imbibed into the channels by capillary forces,whereupon it solidifies and shrinks to form sulphur nanofibres that are in intimate contact with the conductive carbon walls.The scanning electron microscopy (SEM)images in Fig.1reveal the changes in the mixture of CMK-3and sulphur before and after heating.The bulk sulphur evident in the SEM image of the composite on initial mixing (Fig.1a)largely disappears at 145◦C (see Supplementary Fig.S1),and completely disappears after heat treatment at 155◦C (Fig.1b).Full incorporation of sulphur into the channels of CMK-3occurs at this latter temperature.CMK-3and sulphur are both hydrophobic materials,which accounts for the ready absorption of sulphur into the channel structure.The filling of the carbon channels with sulphur is corroborated by the transmission electron microscopy (TEM)image shown in Fig.2a,along with the magnified image shown in Fig.2b.The fibres have a similar diameter to that of the channels of the mesoporous carbon (3.3nm),and a comparable diameter to the carbon nanorods that enclose them (6–7nm).The filling of the pores with sulphur,of similar density to carbon,is also evident from the decrease in contrast in relation to CMK-3itself (shown in the inset in Fig.2b).The sulphur and carbon elemental maps (Fig.2c,d)clearly demonstrate that sulphur is homogeneously distributed in the framework of the mesoporous carbon,with no significant fraction on the external surface.The marked diminution3 nm6.5 nmC Ka1_2S Ka1S meltS xtalx abc def30 nmFigure 2|TEM image and elemental maps of a CMK-3/S-155composite particle and schematic diagrams of the structure and redox processes.a ,CMK-3/S-155composite particle.b ,Image expansion corresponding to the area outlined by the red square in a ,where the inset shows the TEM image for pristine CMK-3at the same magnification.c ,d ,Corresponding carbon and sulphur elemental maps showing the homogeneous distribution of sulphur.e ,A schematic diagram of the sulphur (yellow)confined in the interconnected pore structure of mesoporous carbon,CMK-3,formed from carbon tubes that are propped apart by carbon nanofibres.f ,Schematic diagram of composite synthesis by impregnation of molten sulphur,followed by its densification on crystallization.The lower diagram represents subsequent discharging–charging with Li,illustrating the strategy of pore-filling to tune for volume expansion/contraction.of the X-ray diffraction (XRD)peak (low-angle diffraction pattern,Fig.3a)due to long-range order in CMK-3is further proof of pore-filling,which is the result of the decrease in the scattering contrast (Fig.3a)paring the wide-angle XRD patterns in Fig.3b,the well-resolved peaks corresponding to bulk crystalline sulphur completely disappear after sulphur impregnation,and thermogravimetric analysis (TGA;Supplementary Fig.S2)shows the composites range up to 70wt%sulphur.A schematic diagram illustrating the impregnation of the CMK-3with sulphur is shown in Fig.2e,showing the alignment of the channels in comparison with the inset of Fig.2b.Note that most of the sulphur is contained within the interior of the pore structure,as the particles span hundreds of carbon channels in width.The average CMK-3particle size is of the order of 1µm (Fig.1b).Table 1summarizes the physical characteristics of the CMK-3and the CMK-3/S composite derived from Brunauer–Emmett–Teller (BET)and conductivity measurements.After imbibition of the sulphur in the channels,the pore size of the CMK-3/S composite decreases markedly,indicating that the channels of CMK-3are partially filled.Along with the presence of residual micropores in the carbon wall structure 39,this allows ingress of electrolyte within the structure.Empty volume within the pores is also necessary to accommodate the uptake of Liions,I n t e n s i t y2 (°)I n t e n s i t yi abθ2 (°)θFigure 3|XRD patterns of CMK-3/S before and after heating.a ,Low-angle XRD patterns of a mixture of CMK-3and sulphur before heating (i)and after heating at 155◦C (ii).The disappearance of the first peak is due to the loss of contrast on sulphur imbibition.b ,Wide-angle XRD patterns of a mixture of CMK-3and sulphur before heating (i)and after heating at 155◦C (ii),showing the complete incorporation of crystalline sulphur within the framework.given by the reaction S +2Li →Li 2S,because of the lower density of Li 2S (1.66g cm −3)compared with sulphur.Note that the 70wt%sulphur/composite ratio is less than the theoretical limit of 79wt%sulphur/composite based on the pore volume of CMK-3(2.1cm 3g −1)and the density of liquidized sulphur (1.82g cm −3),and is precisely tuned for the volume expansion (see the Methods section).Using even lower S/carbon ratios provides less ‘stuffed’structures and extra porosity,but at the expense of reduced active mass.Most importantly,the electrical conductivity of the composites (∼0.2S cm −1for 70wt%sulphur/composite)is the same as its mesoporous carbon counterpart.The insulating sulphur merely occupies the empty channels in the mesoporous carbon and does not block the electrical current transporting paths.Three-dimensional,multiple electronic contacts are provided by the numerous carbon interconnects that span the channels,as illustrated schematically in Fig.2e,f (ref.35).Coin cells using a metallic Li anode were assembled to evaluate the materials.All of the capacity values in this article are calculated on the basis of sulphur mass.The first discharge–charge curve for a typical nanostructured CMK-3/S cathode is shown in Fig.1d alongside its SEM image,and is compared with a simple physical (unheated)mixture of 7:3weight ratio of sulphur and CMK-3in Fig.1c.The nanostructured composite exhibits an impressive capacity of 1,005mA h g −1.In contrast,the ‘macro-mixture’exhibited a reversible capacity of 390mA h g −1(on average between 300and 420mA h g −1),similar to that reported in the literature for C–S composites 10.The capacity of CMK-3/S was3.02.52.01.5V o l t a g e (V ) v e r s u s L i +/L i3.02.52.01.5V o l t a g e (V ) v e r s u s L i +/L i3006009001,2001,500Specific capacity (mA h g ¬1)S p e c i f i c c a p a c i t y (m A h g ¬1)CMK¬3/S at 55 °C with C/10 + C/10000.20.40.60.81.01.21.41.6x in Li x S Cycle numberCycle number200406080100S u l p h u r i n e l e c t r o l y t e /t o t a l s u l p h u r (%)1,0001,400abcFigure 4|Electrochemical characterization of PEG-coated CMK-3/S and comparison to reference materials.a ,Lower panel:galvanostaticdischarge–charge profile of PEG-modified CMK-3/S-155recorded at room temperature at 168mA g −1.The reversible capacity of 1,320mA h g −1at room temperature is very close to that obtained for unmodified CMK-3/S obtained at elevated temperature under ‘quasi-equilibrium’conditions shown in the upper panel (CMK-3/S-155recorded at 55◦C at 168mA g −1on discharge to 1.0V followed by quasi-equilibrium discharge at 16.8mA g −1).The slight overcharge in the latter case is due to dissolution of some polysulphide,which is minor even at these conditions.This also indicates that storage of the cell at partial or full discharge does not lead to significant capacity loss.b ,Cycling stability comparison of CMK-3/S-PEG (upper points,in black)versus CMK-3/S (lower points,in red)at 168mA g −1at room temperature.c ,Percentage of sulphur dissolution into the electrolyte from:the CMK-3/S-PEGcomposite cathode (black curve);from the CMK-3/S composite cathode (blue curve);a cathode made of a mixture of acetylene black carbon and sulphur with the exact same C /S ratio (red curve).highly reproducible over many cells.The coulombic efficiency for CMK-3/S in the first discharge–charge cycle is 99.94%without any overcharge,with virtually no irreversibility.This indicates that a very low fraction of polysulphide anions diffuse into the electrolyte.The polarization was decreased by more than a factor of three,owing to the greatly enhanced electrical contact achieved in the nanostructure.Further unequivocal proof of the effectiveness of the contact arises from experiments in which the degree of S incorporation was varied.Nanostructured composites (CMK-3/S-145)with the same S/C ratio,but heated at 145◦C instead of 155◦C result in less complete diffusion of sulphur into the channels because of the higher viscosity at the lower temperature.These composites showed less utilization of sulphur (capacity of 780mA h g −1)in the first discharge sweep (see Supplementary Fig.S3),and an irreversible capacity of 50mA h g −1on plete imbibition prevents sulphur agglomerates on the externalsurface of the mesoporous framework that would have poorer electrical wiring of the conductive carbon phase.These results are superior to those reported for sulphur in contact with multi-walled carbon nanotubes.Such composites exhibit lower capacities and a large electrochemical hysteresis 23.Although the sulphur is apparently confined in the carbon,the contact is limited owing to the relatively large diameter (∼50nm)of the multi-walled carbon nanotubes,and hence of the sulphur fibres within them.Thus,the efficiency of electron transfer to the sulphur mass and accessibility to the Li +electrolyte has a vitally important role in determining the electrochemical behaviour.As seen in Fig.1d,there are two plateaux in the discharge process.The first,which contributes a minor part to the overall capacity from 2.4to 2.0V,corresponds to the conversion from elemental sulphur (S 8)to Li polysulphide anions (Li 2S x ;where x is typically 4–5).The kinetics of this reaction is fast 40.The second plateau atHeat flow (W g ¬1)W e i g h t (%)Temperature (°C)Figure 5|TGA of PEG-modified CMK-3.TGA and differential scanning calorimetry curves recorded in air with a heating rate of 20◦C min −1,for PEG-CMK-3(solid lines),compared with PEG itself (dashed lines),showing the shift to higher temperature of the PEG release on bonding to the CMK-3framework.around 2.0V is due to the conversion of polysulphides to Li 2S 2and then to Li 2S,which occurs at a much slower rate.As we achieve a nominal reversible capacity of Li 1.2S in the nanostructured composite,we wanted to explore the limitations to full conversion.To gain a measure of the reversible capacity under conditions where the kinetics should be a minimal concern,we carried out discharge of the CMK-3/S cathode at 55◦C at 168mA g −1to a cutoff of 1.0V,and allowed the voltage to relax to equilibrium.We then switched the discharge current to a rate of 16.8mA g −1to the end of discharge,and completed charge at 168mA g −1.The electrochemical profile is presented in Fig.4a (upper panel).Under these close-to-equilibrium conditions of full discharge,we achieve a reversible capacity of 1,400mA h g −1—84%of the theoretical capacity (1,675mA h g −1)—indicating that indeed,the kinetics of the last reaction step has a role in capacity limitation.The other factor could be a transport problem.There is progressively more limited accessibility of Li +ions and electrolyte to the sulphur mass towards the end of discharge because the pores become filled with insoluble Li x S (x =1–2)—even though at 70wt%sulphur loading,there is sufficient space for the volume expansion based on the conversion of S to Li 2S.However,we observed that in doubling the rate from 168to 336mA g −1(equivalent to C/5rate),the capacity is reduced by only a small amount to 930mA h g −1(see Supplementary Fig.S4).The mesoporous carbon clearly performs very well as a sulphur container.This is apparent from the small degree of overcharge even under rigorous (55◦C;C/100discharge)conditions as shown in Fig.4a.The complete lack of a sharp minimum in the discharge curve between the two plateaux,as observed by others and ascribed to supersaturation of the electrolyte with S 2−(refs 21,41),is also indicative of the strong extent of sulphide containment in our case.Experiments were carried out to evaluate the degree of self-discharge,by taking the cell to a voltage of 2.1V,holding it at the open-circuit voltage for 24h and then completing discharge.The discharge capacity after relaxation was 5%less than the cell taken to full discharge without the open-circuit voltage step.However,this suggests that the framework still allows for some egress of dissolved sulphur species.We propose that the complex inner pathway and porous,absorptive carbon greatly retard the diffusion of the bulky polysulphide anions out from the channels into the electrolyte,butcannot entirelyprevent it.This is evident by the very slow capacity fading shown in Fig.4b(upperred points).To further trapthe highly polar polysulphide species,we adjusted the hydrophilicity of the carbon external surface afterabcd300 nmFigure 6|Changes in surface morphology of CMK-3/S-155versusPEG-modified CMK-3/S-155on cycling.a ,b ,SEM images of CMK-3/S-155before (a )and after (b )the 15th charge.c ,d ,SEM images of PEG-modified CMK-3/S before (c )and after (d )the 15th charge.Images show the effects of ‘polymer protection’in inhibiting surface deposition.sulphur imbibition by functionalizing the surface with polyethylene glycol (PEG)chains of varying molecular weight.The attachment of the PEG to CMK-3is evident by TGA (Fig.5).The release of the PEG tethered to the CMK-3occurs at 50◦C higher than in PEG itself owing to the ester bonds.The discharge–charge profile of CMK-3/S-PEG is shown in Fig.4a (lower panel).Not only is the initial discharge capacity increased to 1,320mA h g −1(approaching the ‘equilibrium’limit for CMK-3/S of 1,400mA h g −1),and the polarization decreased to low values,but no fading is observed in the second 10cycles and the capacity is stabilized at 1,100mA h g −1on cycling (Fig.4b,upper black points).The entrapment of sulphur active mass on cycling in the polymer-modified CMK-3/S composite is demonstrated in Fig.4c.To measure the degree of sulphur retention in the cathode,a 1.0M LiPF 6solution in a sulphur-free solvent,tetra(ethylene glycol)dimethyl ether (TEGDME),was used as the electrolyte.Glyme solvents are known for their excellent ability to dissolve polysulphides,and hence represent an ‘aggressive’pared with the cathode made of a mixture of sulphur and acetylene black that loses 96%of the total active mass into the electrolyte after 30cycles,the polymer-modified composite shows significant retention of sulphur.Only 25%of the total active mass is solubilized in the electrolyte after 30cycles.The polysulphide retention is also improved in relation to CMK-3/S.We believe that the effect of the PEG-functionalized surface is twofold.First,it serves to trap the polysulphide species by providing a highly hydrophilic surface chemical gradient that preferentially solubilizes them in relation to the electrolyte.Second,by limiting the concentration of the polysulphide anions in the electrolyte,the redox shuttle mechanism is curtailed to a large degree.Deposition of insoluble sulphur species on the surface of the Li electrode and formation of irreversible Li 2S on the cathode surface are strongly inhibited.The last point is clearly demonstrated in SEM images of the PEG-functionalized CMK-3/S cathode before and after cycling,which exhibit very little change in surface morphology (Fig.6),compared with CMK-3/S,which clearly shows precipitation of insoluble products on the surface of the mesoporous carbon particles.In summary,we demonstrate that the strategy illustrated here provides a versatile route to nanostructured polymer-modified mesoporous carbon–sulphur composites that display all of the benefits of confinement effects at a small length scale.Intimate contact of the insulating sulphur and discharge-product sulphides with the retaining conductive carbon framework at nanoscaledimensions affords excellent accessibility of the active material. The carbon framework not only acts as an electronic conduit to the active mass encapsulated within,but also serves as a mini-electrochemical reaction chamber.The entrapment ensures that a more complete redox process takes place,and results in enhanced utilization of the active sulphur material.This is vital to the success of all conversion reactions to ensure full reversibility of the back-reaction.The polymer coating on the external surface of the composite further helps retard diffusion of polysulphide out of the cathode structure,minimize the loss of the active mass in the cathode and improve the cycling stability.The composite materials reported here can supply up to nearly80%of the theoretical capacity of sulphur(1,320mA h g−1),representing more than three times the energy density of lithium transition-metal oxide cathodes,at reasonable rates with good cycling stability.In our laboratory,mesoporous carbon frameworks with various wall thicknesses,conductivities and connectivities have recently been prepared to take advantage of structural and electronic variation of the constraining support.The three-dimensional variants such as CMK-1and CMK-8are particularly promising in this respect42. We will report those results in a forthcoming paper.Owing to the flexibility of the method,the high capacity of the carbon for active material incorporation and facile functionalization of the surface,we believe that a wide variety of nanostructured‘imbibed’composites could find broad application in many areas of materials science,not only as advanced electrode materials that rely on assimilation and conversion reactions.MethodsSynthesis.For the synthesis of SBA-15with controlled morphology43,2g of Pluronic P123(EO20PPO70EO20)was dissolved in60ml of2M HCl at38◦C. Tetraethylorthosilicate(4.2g)was added to the above solution with vigorous stirring.The mixture was stirred for only6min and remained quiescent for24h at38◦C.The mixture was subsequently heated at100◦C for another24h in an autoclave.The as-synthesized SBA-15with short-rod morphology was collected by filtration,dried and calcined at550◦C in air.A nanocasting method was used to fabricate CMK-3from SBA-15as a hard template44.Sucrose(1.25g)was dissolved in5.0ml of water containing0.14g H2SO4.Surfactant-free SBA-15(1.0g)was then dispersed in the above solution and the mixture was sonicated for1h;heated at100◦C for12h and at160◦C for another12h.The impregnation process was repeated once with another5.0ml aqueous solution containing0.8g sucrose and 0.09g H2SO4.The composite was completely carbonized at900◦C for5h in an argon atmosphere.To remove the SBA-15silica template,the composite was stirred in a5%HF solution at room temperature for4h,although NaOH can also be used to dissolve the silica.The CMK-3/S nanocomposite was prepared following a melt-diffusion strategy.CMK-3(1.0g)and sulphur(2.33g)were ground together,and heatedto155◦C.The weight ratio of sulphur/carbon was adjusted to be equal to or less than7:3,to allow for expansion of the pore content on full lithiation to Li2S.For example,1.0g of CMK-3can accommodate3.486g of Li2S(1.66g cm−3(density of Li2S)×2.1cm3g−1,the pore volume of the CMK-3),which corresponds to a maximum of2.425g of sulphur.To prepare the CMK-3/S-PEG composite,CMK-3was first functionalized with carboxylic groups by oxidization treatment in concentrated HNO3solution for half an hour at80◦C,before incorporation of the sulphur.To tether the PEG chains to the surface of the CMK-3/S composite,the composite was dispersedin a PEG aqueous solution and the solution was heated at58◦C and stirred continuously overnight to ensure complete reaction of the carboxylic groups on the carbon particles with the hydroxyl groups on the PEG.The mixture was sonicated for20min to completely remove physically absorbed PEG on the composite,and the CMK-3/S-PEG composite was collected by filtration and dried. Characterization.X-ray diffraction patterns at low-angle(0.75◦to4◦2θ)and wide-angle(from10◦to80◦2θ)were collected on a D8-ADVANCE powderX-ray diffractometer operating at40kV and30mA and using Cu-Kαradiation (λ=0.15406nm).Nitrogen adsorption and desorption isotherms were obtained using a Micromeritics Gemini2735system at−196◦C.Before measurement of CMK-3,the sample was degassed at150◦C on a vacuum line following a standard protocol.It was not possible to carry this out for CMK-3/S owing to the volatility of the sulphur,and so no pretreatment was used.The BET method was usedto calculate the surface area45.The total pore volumes were calculated fromthe amount adsorbed at a relative pressure of0.99.The pore size distributions were calculated by means of the Barrett–Joyner–Halenda method applied to the desorption branch46.As the mesopores of CMK-3/S are decreased to micropores on(partial)filling with sulphur,the possibility of water entrapment,and/or pore blockage means that the values represent lower estimates.The morphology of the sulphur/CMK-3composites were examined by SEM using a LEO1530field-emission SEM instrument or a Hitachi S-5200 instrument.TEM was carried out on a Hitachi HD-2000STEM.Conductivity measurements were carried out at room temperature using the four-point method.Sample bars for the measurement were cut from the pellets and then cold pressed using a force of45kN.Elemental analyses were carried out at M-H-W Laboratories,Phoenix,USA.Electrochemistry.Positive electrodes were comprised84wt%CMK-3/S composite,8wt%Super-S carbon and8wt%poly(vinylidene fluoride)binder. The cathode materials were slurry-cast from cyclopentanone onto a carbon-coated aluminium current collector(Intelicoat).The electrolyte is composed of a1.2M LiPF6solution in ethyl methyl sulphone47.Lithium metal foil was used as the counter electrode.The equivalent current density for the168mA g−1rate is0.19 and0.37mA cm−2for the336mA g−1rate.To measure the degree of sulphur retention in the cathode,a1.0M LiPF6solution in TEGDME was used as the electrolyte.Cathodes comprising CMK-3/S-PEG were compared with simple mixtures of sulphur and acetylene black at the exact same S/C ratio.We used large Swagelok-type cells that accommodate a sufficient excess of the electrolyte to dissolve sulphur species.Swagelok cells were disassembled and immersed into TEGDME to completely extract sulphur species from the electrolyte.Sulphur analysis was carried out by Galbraith Laboratories(Tennessee,USA).Received10September2008;accepted17April2009; published online17May2009References1.Winter,M.&Brodd,R.Batteries,fuel cells and supercapacitors.Chem.Rev.104,4245–4269(2004).2.Bruce,P.G.Energy storage beyond the horizon:Rechargeable lithium batteries.Solid State Ion.179,752–760(2008).3.Rauh,R.D.,Abraham,K.M.,Pearson,G.F.,Surprenant,J.K.&Brummer,S.B.A lithium/dissolved sulfur battery with an organic electrolyte.J.Electrochem.Soc.126,523–527(1979).4.Shim,J.,Striebel,K.A.&Cairns,E.J.The lithium/sulfur rechargeable cell.J.Electrochem.Soc.149,A1321–A1325(2002).5.Kang,K.,Meng,Y.S.,Bréger,J.,Grey,C.P.&Ceder,G.Electrodes withhigh power and high capacity for rechargeable lithium batteries.Science311, 977–980(2006).6.Peled,E.&Yamin,H.Lithium/sulfur organic battery.Prog.Batteries Sol.Cells5,56–58(1984).7.Chu,M.-Y.Rechargeable positive Patent US5686201(1997).8.Peramunage,D.&Licht,S.A solid sulfur cathode for aqueous batteries.Science261,1029–1032(1993).9.Dean,J.A.(ed.)Lange’s Handbook of Chemistry3rd edn,3–5(McGraw-Hill,1985).10.Cunningham,P.T.,Johnson,S.A.&Cairns,E.J.Phase equilibria inlithium–chalcogen systems:Lithium–sulfur.J.Electrochem.Soc.119,1448–1450(1972).11.Choi,J.-W.et al.Rechargeable lithium/sulfur battery with suitable mixedliquid electrolytes.Electrochim.Acta52,2075–2082(2007).12.Rauh,R.D.,Shuker,F.S.,Marston,J.M.&Brummer,S.B.Formationof lithium polysulfides in aprotic media.J.Inorg.Nucl.Chem.39,1761–1766(1977).13.Cheon,S.-E.et al.Rechargeable lithium sulfur battery II.Rate capability andcycle characteristics.J.Electrochem.Soc.150,A800–A805(2003).14.Shin,J.H.&Cairns,E.J.Characterization of N-methyl-N-butylpyrrolidiniumbis(trifluoromethanesulfonyl)imide-LiTFSI-tetra(ethylene glycol)dimethyl ether mixtures as a Li metal cell electrolyte.J.Electrochem.Soc.155,A368–A373(2008).15.Yuan,L.X.et al.Improved dischargeability and reversibility of sulfur cathodein a novel ionic liquid mun.8,610–614(2006).16.Ryu,H.-S.et al.Discharge behavior of lithium/sulfur cell with TEGDME basedelectrolyte at low temperature.J.Power Sources163,201–206(2006).17.Wang,J.et al.Sulfur-mesoporous carbon composites in conjunction with anovel ionic liquid electrolyte for lithium rechargeable batteries.Carbon46, 229–235(2008).18.Chung,K.-I.,Kim,W.-S.&Choi,Y.-K.Lithium phosphorous oxynitride as apassive layer for anodes in lithium secondary batteries.J.Electroanal.Chem.566,263–267(2004).19.Visco,S.J.,Nimon,Y.S.&Katz,B.D.Ionically conductive composites forprotection of active metal Patent7,282,296,October16(2007). 20.Skotheim,T.A.,Sheehan,C.J.,Mikhaylik,Y.V.&Affinito,J.Lithium anodesfor electrochemical patent7247,408,July24(2007).21.Akridge,J.R.,Mikhaylik,Y.V.&White,N.Li/S fundamental chemistry andapplication to high-performance rechargeable batteries.Solid State Ion.175, 243–245(2004).。
纳米技术ppt
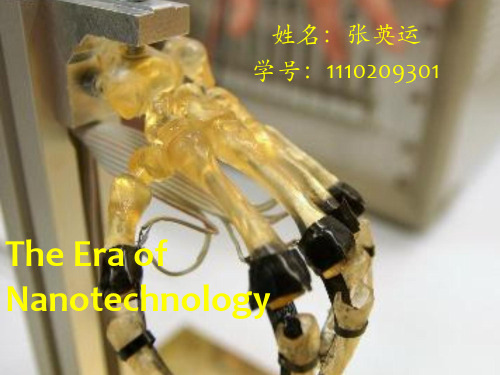
Scanning electron microscope
SEM (SEM) scanning electron microscope is a type of microscope that images a sample by scanning it with a high-energy beam of electrons in a raster scan pattern. The electrons interact with the atoms that make up the sample producing signals that contain information about the sample's surface topography, composition, and other properties such as electrical conductivity. The types of signals produced by an SEM include secondary electrons, back-scattered electrons (BSE), characteristic X-rays, light (cathodoluminescence),
Current image of C
60
Procedure of STM
In constant height mode, the voltage and height are both held constant while the current changes to keep the voltage from changing; this leads to an image made of current changes over the surface, which can be related to charge density. The benefit to using a constant height mode is that it is faster, as the piezoelectric movements require more time to register the change in constant current mode than the voltage response in constant height mode. All images produced by STM are grayscale, with color optionally added in post-processing in order to visually emphasize important features. In addition to scanning across the sample, information on the electronic structure at a given location in the sample can be obtained by sweeping voltage and measuring current at a specific location. This type of measurement is called scanning tunneling spectroscopy (STS) and typically results in a plot of the local density of states as a function of energy within the sample. The advantage of STM over other measurements of the density of states lies in its ability to make extremely local measurements: for example, the density of states at an impurity site can be compared to the density of states far from impurities.
Li4Ti5O12的孔状微纳米结构对实验有所帮助
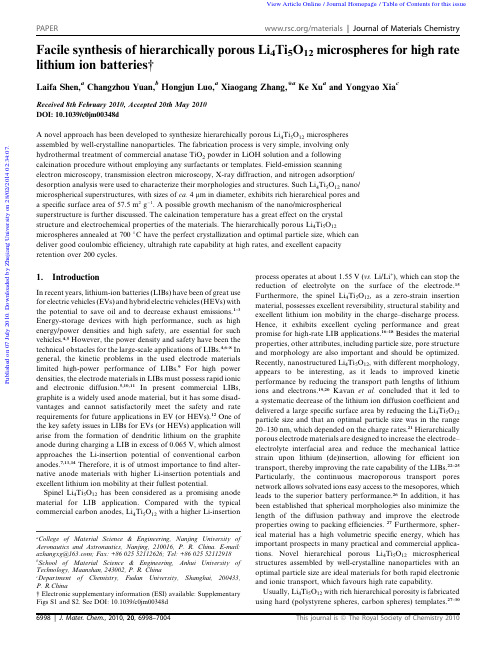
Facile synthesis of hierarchically porous Li 4Ti 5O 12microspheres for high rate lithium ion batteries †Laifa Shen,a Changzhou Yuan,b Hongjun Luo,a Xiaogang Zhang,*a Ke Xu a and Yongyao Xia cReceived 8th February 2010,Accepted 20th May 2010DOI:10.1039/c0jm00348dA novel approach has been developed to synthesize hierarchically porous Li 4Ti 5O 12microspheres assembled by well-crystalline nanoparticles.The fabrication process is very simple,involving only hydrothermal treatment of commercial anatase TiO 2powder in LiOH solution and a following calcination procedure without employing any surfactants or templates.Field-emission scanning electron microscopy,transmission electron microscopy,X-ray diffraction,and nitrogen adsorption/desorption analysis were used to characterize their morphologies and structures.Such Li 4Ti 5O 12nano/microspherical superstructures,with sizes of ca.4m m in diameter,exhibits rich hierarchical pores and a specific surface area of 57.5m 2g À1.A possible growth mechanism of the nano/microspherical superstructure is further discussed.The calcination temperature has a great effect on the crystal structure and electrochemical properties of the materials.The hierarchically porous Li 4Ti 5O 12microspheres annealed at 700 C have the perfect crystallization and optimal particle size,which can deliver good coulombic efficiency,ultrahigh rate capability at high rates,and excellent capacity retention over 200cycles.1.IntroductionIn recent years,lithium-ion batteries (LIBs)have been of great use for electric vehicles (EVs)and hybrid electric vehicles (HEVs)with the potential to save oil and to decrease exhaust emissions.1–3Energy-storage devices with high performance,such as high energy/power densities and high safety,are essential for such vehicles.4,5However,the power density and safety have been the technical obstacles for the large-scale applications of LIBs.4,6–8In general,the kinetic problems in the used electrode materials limited high-power performance of LIBs.9For high power densities,the electrode materials in LIBs must possess rapid ionic and electronic diffusion.5,10,11In present commercial LIBs,graphite is a widely used anode material,but it has some disad-vantages and cannot satisfactorily meet the safety and rate requirements for future applications in EV (or HEVs).12One of the key safety issues in LIBs for EVs (or HEVs)application will arise from the formation of dendritic lithium on the graphite anode during charging a LIB in excess of 0.065V,which almost approaches the Li-insertion potential of conventional carbon anodes.7,13,14Therefore,it is of utmost importance to find alter-native anode materials with higher Li-insertion potentials and excellent lithium ion mobility at their fullest potential.Spinel Li 4Ti 5O 12has been considered as a promising anode material for LIB pared with the typical commercial carbon anodes,Li 4Ti 5O 12with a higher Li-insertionprocess operates at about 1.55V (vs.Li/Li +),which can stop the reduction of electrolyte on the surface of the electrode.15Furthermore,the spinel Li 4Ti 5O 12,as a zero-strain insertion material,possesses excellent reversibility,structural stability and excellent lithium ion mobility in the charge–discharge process.Hence,it exhibits excellent cycling performance and great promise for high-rate LIB applications.16–18Besides the material properties,other attributes,including particle size,pore structure and morphology are also important and should be optimized.Recently,nanostructured Li 4Ti 5O 12,with different morphology,appears to be interesting,as it leads to improved kinetic performance by reducing the transport path lengths of lithium ions and electrons.19,20Kavan et al.concluded that it led to a systematic decrease of the lithium ion diffusion coefficient and delivered a large specific surface area by reducing the Li 4Ti 5O 12particle size and that an optimal particle size was in the range 20–130nm,which depended on the charge rates.21Hierarchically porous electrode materials are designed to increase the electrode–electrolyte interfacial area and reduce the mechanical lattice strain upon lithium (de)insertion,allowing for efficient ion transport,thereby improving the rate capability of the LIBs.22–25Particularly,the continuous macroporous transport pores network allows solvated ions easy access to the mesopores,which leads to the superior battery performance.26In addition,it has been established that spherical morphologies also minimize the length of the diffusion pathway and improve the electrode properties owing to packing efficiencies.27Furthermore,spher-ical material has a high volumetric specific energy,which has important prospects in many practical and commercial applica-tions.Novel hierarchical porous Li 4Ti 5O 12microspherical structures assembled by well-crystalline nanoparticles with an optimal particle size are ideal materials for both rapid electronic and ionic transport,which favours high rate capability.Usually,Li 4Ti 5O 12with rich hierarchical porosity is fabricated using hard (polystyrene spheres,carbon spheres)templates.27–30aCollege of Material Science &Engineering,Nanjing University of Aeronautics and Astronautics,Nanjing,210016,P.R.China.E-mail:azhangxg@;Fax:+8602552112626;Tel:+8602552112918bSchool of Material Science &Engineering,Anhui University of Technology,Maanshan,243002,P.R.China cDepartment of Chemistry,Fudan University,Shanghai,200433,P.R.China†Electronic supplementary information (ESI)available:Supplementary Figs S1and S2.See DOI:10.1039/c0jm00348dPAPER /materials |Journal of Materials ChemistryP u b l i s h e d o n 07 J u l y 2010. D o w n l o a d e d b y Z h e j i a n g U n i v e r s i t y o n 28/02/2014 02:34:07.View Article Online / Journal Homepage / Table of Contents for this issueHowever,all the suggested approaches require a template or structure-directing agent,which increase the production cost and complexity.Therefore,an easily controlled method obviating the need for templates to prepare hierarchical Li 4Ti 5O 12with rich hierarchical porosity is still a big challenge for researchers.In this work,we report a simple,template-free,hydrothermal method for the production of Li 4Ti 5O 12hierarchical micro-spheres assembled by uniform nanoparticles.To the best of our knowledge,it is the first report on one step hydrothermal synthesis of Li 4Ti 5O 12hierarchical microspheres with micro-and nano-scale composite structures.This method is very facile and effective for preparing the microsphere materials in high yield and with uniform diameter.On the basis of the crystal structures and morphology’s evolution with time,the possible mechanism for the formation of the Li 4Ti 5O 12microspheres is also presented and discussed.The electrochemical properties of as-prepared Li 4Ti 5O 12hierarchical microspheres were investigated as an anode material for LIBs,presenting an excellent high-rate performance and good electrochemical stability.2.Experimental2.1.Hydrothermal synthesis of spinel Li 4Ti 5O 12hierarchical spheresAll of the reactants and solvents were of analytical grade and used without further purification.The spinel Li 4Ti 5O 12micro-spheres were hydrothermally synthesized by using commercial crystalline anatase titania powders (with an average size of 10nm)as raw materials.In a typical synthesis,a stoichiometric amount of anatase titania powder (0.4g)was dispersed in LiOH aqueous solution (60ml,1.0g LiOH $H 2O).After stirring for 10min,the suspension was transferred into an 80mL Teflon-lined autoclave and kept at 180 C for different times.The white precipitate was separated by filtration,washed several times with deionized water to remove the excess hydroxides before drying at 80 C for 6h.Subsequently,the white powder was calcinated at 500–700 C for 2h in air to obtain Li 4Ti 5O 12materials.2.2.CharacterizationThe crystal structure of the obtained samples was characterized by X-ray diffraction (XRD)(Bruker D8advance)with Cu K a radiation.The microstructural properties were obtained using transmission electron microscopy (TEM)(TEM,FEI,Tecnai-20)and high-resolution transmission electron microscopy (HRTEM,JEOL JEM-2010),and field emission scanning electron micros-copy (FESEM,LEO 1430VP).The N 2adsorption/desorption tests were determined by Brunauer–Emmett–Teller (BET)measurements using an ASAP-2010surface area analyzer.2.3.Electrochemical measurementsElectrochemical characterization was carried out by galvano-static cycling in a CR2016-type coin cell.The working electrodes were prepared by a slurry coating procedure.The slurry con-sisted of 80wt%active material,12wt%acetylene black and 8wt%polyvinylidene fluoride (PVDF)dissolved in N -methyl pyrrolidinone (NMP),and was uniformly spread on an aluminium foil current collector.Finally,the electrode was driedunder vacuum at 110 C for 12h.Test cells were assembled in an argon-filled glove box using Li foil as the counter electrode and polypropylene (PP)film as the separator.1mol L À1LiPF 6solution in a 1:1(v :v)mixture of ethylene carbonate (EC)and dimethyl carbonate (DMC)was used as the electrolyte.The coin cells were galvanostatically charged–discharged at different current densities between 1.0and 2.5V (vs Li/Li +)using a CT2001A cell test instrument (LAND Electronic Co.).3.Results and discussion3.1Physicochemical characterizationThe Li 4Ti 5O 12precursor obtained from hydrothermal treatment of anatase titanium dioxide powders in LiOH is a ternary Li–Ti–O phase,the crystal structure of which is still controversial.The intermediate phase is usually assigned to rock salt-type LiTiO 2because the XRD pattern approximately matches its standard JCPDS Card (NO.16-0223).31However,according to our and other work,32,33it is more reasonable that the intermediate phase we obtained here is a LiTi 2O 4+d phase.The XRD pattern (Fig.1a)of the intermediate material we obtained is different from the standard LiTiO 2.Moreover,the white colour of the intermediate material indicates that the Li 4Ti 5O 12precursor contains only tetravalent Ti.33The intermediate phase was cal-cinated at a range of temperatures (500,600and 700 C)to obtain different Li 4Ti 5O 12samples.In the three prepared samples,the main diffraction peaks in their XRD patterns (Fig.1b,c and d)can be indexed to the cubic spinel structure,Li 4Ti 5O 12(JDPDS NO.26-1198).Some small peaks can also be observed near the main peaks of Li 4Ti 5O 12,which can be attributed to Li 2TiO 3(JCPDS NO.08-0249).Notably,the diffraction peaks of Li 4Ti 5O 12gradually sharpened as the sin-tering temperature increased from 500to 700 C,which indicates an increase of crystallinity with the calcination temperature due to the ordering of the local structure and/or release of thelatticeFig.1XRD patterns of the intermediate phase obtained by hydro-thermal treatment for 24h in LiOH solution (a)and Li 4Ti 5O 12samples synthesized by calcinating the intermediate phase at 500 C (b),600 C (c)and 700 C (d).P u b l i s h e d o n 07 J u l y 2010. D o w n l o a d e d b y Z h e j i a n g U n i v e r s i t y o n 28/02/2014 02:34:07.strain.By using Scherrer’s formula based on the (111)peak,the grain size of Li 4Ti 5O 12is estimated to be 11.2,18.7,and 32.9nm for 500 C,600 C,and 700 C,respectively,which confirms the growth of Li 4Ti 5O 12nanoparticles.The morphology of the synthesized samples was observed by FESEM.The intermediate phase is clearly spherical with a diameter of 4m m,as can be seen in Fig.2a.Upon higher resolution magnification (Fig.2b),the product is assembled by many fine nanoparticles and its surface is rough.The pore structure consists of a wormhole-like pore array,and the wall of the hierarchical framework consists of interconnected nano-particles.From Fig.2c,e and g,the overall morphology of the particles has been well maintained after calcinations.It is worth noting that during the annealing process at different tempera-tures,the size of the Li 4Ti 5O 12microspheres was maintained,while the grain size of nanoparticles increases with the increase of annealing temperature from 500to 700 C (Fig.2d,f and h),which is consistent with the XRD analysis above.Macropores or large mesopores formed among the nanoparticles,and the mes-opores among the small primary particles produced a hierar-chical porous structure in the crystalline Li 4Ti 5O 12microspheres.The porous structure becomes more apparent with the increase of the annealing temperature.Obviously,all the productsconfirm the hierarchical 3D nano/micro superstructures assem-bled by the different sizes of the nanoparticles.It is expected that such a combination will enhance the porosity of the electrode material,which in turn plays a vital role in enhancing the power density via reducing the diffusion path length for ionic and electronic mobility.34To further examine the architecture of the Li 4Ti 5O 12micro-spheres,the samples were investigated by TEM and HRTEM.Fig.3a shows a representative monodispersed Li 4Ti 5O 12micro-sphere obtained after calcination at 700 C.Its diameter is ca.4m m,in good agreement with the SEM,shown in Fig.2g.As shown in Fig.3(b and c),the Li 4Ti 5O 12microsphere’s structure is actually constructed from the assembly of interconnected nanoparticles with a diameter of ca.33nm.The crystallite sizes are in good agreement with that estimated from XRD.Further evidence for the porous structure can also be found from Fig.3(b and c).Many hierarchical pores,in sizes in the range 10–50nm,formed among the fine primary particles in the spheres of Li 4Ti 5O 12,are clearly seen in the images.Mesoporosity in the present system may arise from an interconnected three-dimen-sional network generating a mesoporous configuration.Fig.2d shows that the interplanar distance between adjacent lattice planes is 0.48nm,corresponding to the (111)lattice fringe of spinel Li 4Ti 5O 12,which indicates the prepared Li 4Ti 5O 12hier-archical microspheres are assembled by the well-crystallized spinel Li 4Ti 5O 12phase nanoparticles.30To gain further insight into the porous structure and pore size distribution of the Li 4Ti 5O 12nano/micro superstructure,Bru-nauer–Emmett–Teller (BET)measurements were performed to examine its specific textural properties.As shown in Fig.4a,the nitrogen adsorption–desorption isotherm can be attributed to type IV,according to IUPAC classification.The hierarchical assembly of Li 4Ti 5O 12microspheres shows a distinct hysteresis in the larger range of ca.0.7–1.0P/P 0,indicating the presence of mesopores and macropores possibly formed by the porous stacking of component nanoparticles.35–37The corresponding Barrett–Joyner–Halenda (BJH)pore size distributioncurveFig.2FESEM images of the intermediate phase obtained by hydro-thermal treatment for 24h in LiOH solution (a,b)and Li 4Ti 5O 12samples synthesized by calcinating the intermediate phase at 500 C (c,d),600 C (e,f)and 700 C (g,h).Fig.3TEM (a–c)and HRTEM (d)images of hierarchical porous Li 4Ti 5O 12microspheres obtained at 700 C.P u b l i s h e d o n 07 J u l y 2010. D o w n l o a d e d b y Z h e j i a n g U n i v e r s i t y o n 28/02/2014 02:34:07.(Fig.4b)shows that the pore size is not uniform,the maximum pore diameter and hierarchical distribution is 30.3nm and 2.6nm within the range of the mesopores (2–50nm).Besides,the samples exhibit macropores with pore sizes of up to 100nm.Macropores do not offer as high surface areas,however,they do provide good electrolyte access.The rough surfaces of the product,as well as the interspaces between the fine particles in the FESEM images (Fig.2)and TEM images (Fig.3),also reflect the presence of the hierarchical structure.Moreover,the textural properties of the Li 4Ti 5O 12microspheres are quantitatively shown as follows:the BET specific surface area is 57.5m 2g À1including the micro area of 15.2m 2g À1and the external surface area of 42.3m 2g À1,the mesoporous volume is 0.32cm 3g À1and the average pore size is 22.7nm.Such a porous structure provides the efficient transport pathways to their interior voids and increases the electrode–electrolyte interfacial area,which is crit-ical for high rate LIB applications.3.2Formation of the hierarchical Li 4Ti 5O 12microspheres In order to investigate the formation mechanism of the hierar-chical Li 4Ti 5O 12microspheres,time-dependent experiments were carefully conducted.First,the samples with different hydro-thermal treatment times were investigated by XRD.As shown in Fig.5a,the anatase TiO 2phase (JCPDS NO.21-1207)can still be detected in the XRD pattern of the product after just hydro-thermal treatment for 3h,following calcination at 700 C for 2h.From Fig.5b,it can be found that the typical diffraction peaks of anatase TiO 2disappears while the Li 2TiO 3phase appears after 6h reaction,which indicates that the starting anatase TiO 2was completely converted into a ternary Li–Ti–O phase after chem-ical lithiation.During the following calcination process,the spinel Li 4Ti 5O 12accompanied with a small amount of Li 2TiO 3was formed.The diffraction peaks of the XRD patterns did not change any more,even with longer hydrothermal treatment,as can be seen from Fig.5c.Obviously,there is a ‘‘phase trans-formation’’in the formation process of Li 4Ti 5O 12.To investigate the growth process of the Li 4Ti 5O 12hierarchical microspheres constructed from the assembly of interconnected nanoparticles,SEM images of the intermediate phase obtained from hydro-thermal treatment at different times are presented in Fig.6.The primary TiO 2sample consists of nanoparticles (the SEM image of TiO 2powder is shown in Fig.S1in the ESI†).As shown inFig.6a,when the reaction time was just 3h,the morphology of the sample did not change much.When the reaction time is prolonged to 6h,some nanoparticles aggregated and aligned into sphere-like frames,coexisting with the nanoparticles (Fig.6b).The microsphere’s superstructure appeared as the result of self-assembly of nanoparticles after the reaction time was further prolonged to 12h (Fig.6c).On the basis of SEM,crystal structure and chemical compo-sition analysis,we propose a dissolution–crystallization–assembly mechanism to illustrate the nucleation and growth procedures of Li 4Ti 5O 12(presented in Scheme 1).During the hydrothermal treatment of TiO 2powder in LiOH solution,OH Àand H 2O would enter into the titania nanoparticles and interact with the titanium ions and/or lattice oxygen to form titanium hydroxyl species (probably HTiO 3À).38Then,HTiO 3Àreacted with Li +to form supersaturating particles and precipitate on the TiO x surface.The presence of abundant OH Àwould promote the fast formation of HTiO 3À.This produces a continuous layer of Li–Ti–O,through which additional lithium must diffuse for the reaction to continue,until the TiO 2supply is exhausted.In the above reaction process,the pore formation by the diffusion of HTiO 3Àthrough the shell was balanced by the opposite flow of vacancies to the vicinity of the TiO 2surfaces.This is the reason why the maximum pore size at 2.6nm in the Barrett–Joyner–Halenda pore size distribution curve exists.While theLi–Ti–OFig.4The gas (N 2)adsorption–desorption isotherm loop (a)and a histogram of the pore size distribution data (b)for the synthesized Li 4Ti 5O 12microspheres obtained by calcinating precursor at 700C.Fig.5XRD patterns of the products obtained by calcinating the intermediate phase at 700 C after hydrothermal treatment for 3h (a),6h (b)and 12h (c),respectively.P u b l i s h e d o n 07 J u l y 2010. D o w n l o a d e d b y Z h e j i a n g U n i v e r s i t y o n 28/02/2014 02:34:07.nanoparticles with quite high surface energy in LiOH solution,these monodispersed nanoparticles tend to aggregate to decrease the surface energy by reducing the exposed areas.32,39Therefore,with further hydrothermal treatment for 6h,a spontaneous energy-minimizing self-organization of the resulting intermediate Li–Ti–O particles would occur to remove the surface energy associated with unsatisfied bonds.As a result,the nanoparticles-based Li–Ti–O hierarchical microspheres form through the self-assembly growth mechanism,as mentioned above.Finally,the Li 4Ti 5O 12microspheres were obtained by a short post-annealing of the Li–Ti–O phase.3.2Electrochemical analysisThe Li 4Ti 5O 12samples obtained at the different annealedtemperatures were characterized by constant current charge–discharge tests at a rate of 1C between 1.0and 2.5V.Fig.7displays the typical charge–discharge curves.The sample annealed at 700 C gives a discharge capacity of 162.4mA h g À1,which is the highest among the three samples.The sample obtained at 600 C had an initial discharge capacity of 160.7mA h g À1,a much smaller capacity discharged at a constant voltage.The sample obtained at 500 C achieves a similar discharge capacity to the sample obtained at 600 C,but its coulombic efficiency in the first cycle is the lowest (ca.90.1%),which might be ascribed to the low crystallinity and smaller particle size.The irreversible capacity loss is more pronounced for high surface lithium storage and related to changes in solubility limits due to strain and interface energy,which is also found in other reports.40Moreover,the sample prepared at 700 C shows a very flat charge–discharge plateau at a potential of around 1.55V (vs Li/Li +),which indicates a two-phase reaction:41Li 4Ti 5O 12þ3Li þþ3e À ! dischargecharge Li 7Ti 5O 12However,samples prepared at low temperatures,such as 500and 600 C,deliver sloping plateau charge–discharge curves.Also,the voltage profiles of the samples prepared at low temperatures exhibited a relatively higher charging plateau and lower discharging plateau compared to the 700 C sample.The difference in the charge–discharge profile is mostly correlative to its crystal structure.The formation of the unstable spinel struc-ture at low temperature resulted in low electrochemical reac-tivity.17The crystallinity and size of Li 4Ti 5O 12nanoparticles increase with the increase of calcination temperature,resulting in enhanced charge–discharge capacities and an even flatter potential plateau.It is an effective way to improve the electro-chemical performance of Li 4Ti 5O 12anode materials by synthe-sizing electroactive materials with a perfect crystallization degree and moderate particle size.Since it has delivered an excellent electrochemical property,the hierarchical porous Li 4Ti 5O 12microspheres prepared at 700 C was chosen for further inves-tigation for high power LIB applications.In general,the microstructures assembled by nanoparticles have good rate performances because of short lithium ion diffusion distances and facile electron transport.The Li 4Ti 5O12Fig.6SEM images of the intermediate phase after hydrothermal treatment for 3h (a),6h (b)and 12h (c),respectively.Scheme 1The formation process of the nano/micro Li 4Ti 5O 12micro-spheres.Fig.7Charge–discharge profiles of the Li 4Ti 5O 12samples calcined at 500–700 C at 1C.P u b l i s h e d o n 07 J u l y 2010. D o w n l o a d e d b y Z h e j i a n g U n i v e r s i t y o n 28/02/2014 02:34:07.microspheres prepared at 700 C exhibit good rate capability.Fig.8shows the initial charge–discharge curves of the Li 4Ti 5O 12microspheres at different current rates from 0.2to 20C.At the initial lower rate of 0.2C,the Li 4Ti 5O 12sample gives a discharge capacity of 168.1mA h g À1,which is very close to its theoretical capacity of 175mA h g À1.It should be pointed out that the coulombic efficiency (ca.95%)in the first cycle might be ascribed to the perfect crystallization degree and moderate particle size,which decrease the irreversible capacity.With increasing of the charge–discharge rate,the variations of discharge plateau potential are very small,even up to a high rate of 20C,although the overpotential can be observed.The obtained Li 4Ti 5O 12microspheres show outstanding rate performance (Fig.9).The cells was progressively charged–dis-charged in series stages with the charge–discharge rate from 0.2to 20C.For each stage,the process was taken with 5cycles.A specific discharge capacity is around 165.8mA h g À1obtained at a rate of 0.5C,which is very close to the rate of 0.2C.The specific discharge capacity was slightly reduced to 162.4,156.8,143.9,134.6and 116mA h g À1at rates of 1C,2C,3C,5C and 10C,respectively.At the high rate of 20C,the specific charge capacity is still 92.3mA h g À1.The nano/micro spherical Li 4Ti 5O 12superstructures exhibited a remarkable cycling and rate capa-bility behaviour,which is even superior to the performance reported for Li 4Ti 5O 12nanorods,12Li 4Ti 5O 12nanotubes,16Li 4Ti 5O 12nanowires,19and Li 4Ti 5O 12nanoparticles,42the main reason for which should be that the nano-sized building blocks result in excellent Li insertion–extraction performance.These rich and hierarchical diffusion channels ensure enough lithium ions to rapidly contact the much larger surfaces of the electro-active Li 4Ti 5O 12microspheres.As mentioned earlier,the large surface area introduced by the highly porous nano-structured building blocks of each Li 4Ti 5O 12sphere favoured the creation of an easy and shorter diffusion pathway for ionic and electronic diffusion,resulting in extremely good power performance.Above 40cycles,the Li 4Ti 5O 12electrode was further charged–discharged at 2C for another 200cycles to investigate the cycling performance (see Fig.9).The as-prepared Li 4Ti 5O 12micro-spheres exhibited an excellent cycling performance.The discharge capacity in the first cycle was 154.5mA h g À1,and after200charge–discharge cycles,the capacity remained at 147.4mA h g À1,which was less than 4.8%discharge capacity loss.Calcu-lated from the discharge capacity/charge capacity,the coulombic efficiency in this long cycle period almost kept constant at about 100%(shown in the inset of Fig.10).The excellent cycling ability can be attributed to the good crystallinity of Li 4Ti 5O 12micro-spheres assembled by the optimized particle,which possess the stability of the spinel crystal structure and exhibit a zero-volume expansion over the whole stoichiometric range.4.ConclusionsIn summary,we have developed a facile template-free approach to fabricate Li 4Ti 5O 12microspheres consisting of interconnected Li 4Ti 5O 12nanoparticles,and exhibiting large hierarchical (meso -and macro-)porosity of various sizes,which facilitate the electrons and lithium ion diffusion.The approach involves hydrothermal treatment of anatase TiO 2powder in LiOH solu-tion to form an intermediate Li–Ti–O precursor andfollowingFig.8Galvanostatic charge–discharge curves for the hierarchical Li 4Ti 5O 12spheres annealed at 700 C between 1.0and 2.5V at different currentdensities.Fig.9The rate performance of the hierarchical Li 4Ti 5O 12spheres annealed at 700C.Fig.10The cycling profile of the hierarchical Li 4Ti 5O 12microspheres annealed at 700 C at a rate of 2C.P u b l i s h e d o n 07 J u l y 2010. D o w n l o a d e d b y Z h e j i a n g U n i v e r s i t y o n 28/02/2014 02:34:07.heat treatment at different temperatures.The formation of such unique hierarchical pore Li 4Ti 5O 12microspheres can be ascribed to the dissolution–crystallization–assembly mechanism,and the schematic illustration of the whole formation mechanism was proposed.When tested for electrochemical lithium storage,the hierarchically porous Li 4Ti 5O 12microspheres prepared at 700 C with a perfect crystallization degree and optimal particle size exhibits very constant coulombic efficiency,ultra high rate capability at higher rates,and excellent capacity retention over 200cycles.We believe that such a unique Li 4Ti 5O 12microsphere superstructure has considerable potential as an anode material for high rate lithium ion batteries or hybrid battery capacitors.AcknowledgementsThis work was supported by the National Basic Research Program of China (973Program)(no.2007CB209703)and the National Natural Science Foundation of China (no.206033040,No.20873064).References1M.J.Armand and M.Tarascon,Nature ,2008,451,652–657.2B.Scrosati,Nature ,1995,373,557–558.3M.Tarascon and M.J.Armand,Nature ,2001,414,359–367.4X.L.Wu,L.Y.Jiang,F.F.Cao,Y.G.Guo and L.J.Wan,Adv.Mater.,2009,21,2710–2714.5J.M.Tarascon,Nat.Nanotechnol.,2009,4,341–342.6H.Joachin,T.D.Kaun,K.Zaghib and J.Prakasha,J.Electrochem.Soc.,2009,156,A401–406.7Y.G.Wang,H.M.Liu,K.X.Wang,H.Eiji,Y.R.Wang and H.S.Zhou,J.Mater.Chem.,2009,19,6789–6795.8G.Armstrong, A.R.Armstrong,P.G.Bruce,P.Reale and B.Scrosati,Adv.Mater.,2006,18,2597–2600.9Y.G.Guo,Y.S.Hu,W.Sigle and J.Maier,Adv.Mater.,2007,19,2087–2091.10Y.S.Hu,Y.G.Guo,R.Dominko,M.Gaberscek,J.Jamnik and J.Maier,Adv.Mater.,2007,19,1963–1966.11Y.Wang and G.Z.Cao,Adv.Mater.,2008,20,2251–2269.12Y.F.Tang,L.Yang,S.H.Fang and Z.Qiu,Electrochim.Acta ,2009,54,6244–6249.13T.Kar,J.Pattanayak and S.Scheiner,J.Phys.Chem.A ,2001,105,10397–10403.14K.S.Park,A.Benayad,D.J.Kang and S.G.Doo,J.Am.Chem.Soc.,2008,130,14930–14931.15G.G.Amatucci, F.Badway, A. D.Pasquier and T.Zheng,J.Electrochem.Soc.,2001,148,A930–A939.16S.C.Lee,S.M.Lee,J.W.Lee,J.B.Lee,S.M.Lee,S.S.Han,H.C.Lee and H.J.Kim,J.Phys.Chem.C ,2009,113,18420–18423.17L.Cheng,H.J.Liu,J.J.Zhang,H.M.Xiong and Y.Y.Xia,J.Electrochem.Soc.,2006,153,A1472–A1477.18J.Kim and J.Cho,Electrochem.Solid-State Lett.,2007,10,A81–A81.19J.R.Li,Z.L.Tang and Z.T.Zhang,mun.,2005,7,894–899.20L.Cheng,J.Yan,G.N.Zhu,J.Y.Luo,C.X.Wang and Y.Y.Xia,J.Mater.Chem.,2010,20,595–602.21L.Kavan,J.Prochazka,T.M.Spitler,M.Kalbac,M.T.Zukalova,T.Drezen and M.Gratzel,J.Electrochem.Soc.,2003,150,A1000–A1007.22C.M.Doherty,R.A.Caruso,B.M.Smarsly and C.J.Drummond,Chem.Mater.,2009,21,2895–2903.23H.Yang,X.L.Wu,M.H.Cao and Y.G.Guo,J.Phys.Chem.C ,2009,113,3345–3351.24J.Y.Baek,H.W.Ha,I.Y.Kim and S.J.Hwang,J.Phys.Chem.C ,2009,113,17392–17398.25S.Y.Gao,S.X.Yang,J.Shu,S.X.Zhang,Z.D.Li and K.Jiang,J.Phys.Chem.C ,2008,112,19324–19328.26C.M.Doherty,R.A.Caruso,B.M.Smarsly,P.Adelhelm and C.J.Drummond,Chem.Mater.,2009,21,5300–5306.27E.M.Sorensen,S.J.Barry,H.K.Jung,J.R.Rondinelli,J.T.Vaughey and K.R.Poeppelmeier,Chem.Mater.,2006,18,482–489.28C.H.Jiang,Y.Zhou,I.Honma,T.Kudo and H.S.Zhou,J.Power Sources ,2007,166,514–518.29S.W.Woo,K.Dokko and K.Kanamura,Electrochim.Acta ,2007,53,79–82.30Y.F.Tang,L.Yang,Z.Qiu and J.S.Huang,J.Mater.Chem.,2009,19,5980–5984.31Y.Li,G.L.Pan,J.W.Liu and X.P.Gao,J.Electrochem.Soc.,2009,156,A495–A499.32C.H.Jiang,E.Hosono,M.Ichihara,I.Honma and H.S.Zhou,J.Electrochem.Soc.,2008,155,A553–556.33D.Fattakhova,V.Petrykin,J.Brus,T.Kostlanova,J.Dedecek and P.Krtil,Solid State Ionics ,2005,176,1877–1885.34H.G.Jung,S.W.Oh,C.Jin,N.Jayaprakash and Y.K.Sun,mun.,2009,11,756–759.35C.Z.Yuan,X.G.Zhang,L.H.Su,B.Gao and L.F.Shen,J.Mater.Chem.,2009,19,5772–5777.36J.Y.Baek,H.W.Ha,I.Y.Kim and S.J.Hwang,J.Phys.Chem.C ,2009,113,17392–17398.37X.F.Zhou,Z.L.Hu,Y.Q.Fan,S.Chen,W.P.Ding and N.P.Xu,J.Phys.Chem.C ,2008,112,11722–11728.38Y.W.Wang,H.Xu,X.B.Wang,X.Zhang,H.M.Jia,L.Z.Zhang and J.R.Qiu,J.Phys.Chem.B ,2006,110,13835–13840.39R.L.Penn and J.F.Banfield,Science ,1998,281,969–971.40W.J.H.Borghols,M.Wagemaker,font,E.M.Kelder and F.M.Mulder,J.Am.Chem.Soc.,2009,131,17786–17792.41S.Scharner,W.Weppner and P.Schmid-Beurmann,J.Electrochem.Soc.,1999,146,857–861.42D.H.Kim,Y.S.Ahn and J.Kim,mun.,2005,7,1340–1344.P u b l i s h e d o n 07 J u l y 2010. D o w n l o a d e d b y Z h e j i a n g U n i v e r s i t y o n 28/02/2014 02:34:07.。
科技英语翻译
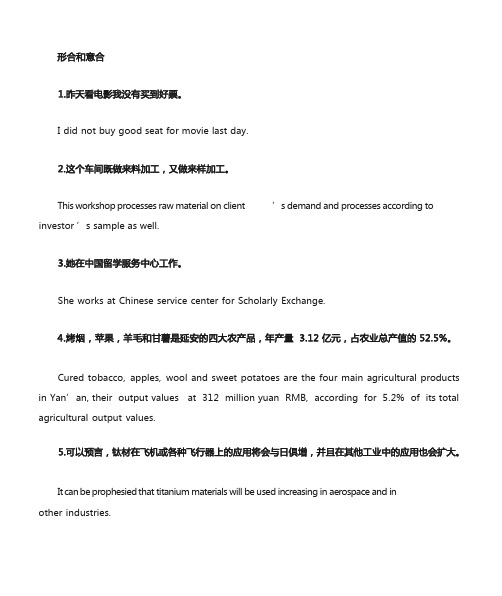
I did not buy good seat for movie last day.This workshop processes raw material on client ’s demand and processes according to investor ’s sample as well.She works at Chinese service center for Scholarly Exchange.Cured tobacco, apples, wool and sweet potatoes are the four main agricultural products in Yan’an, their output values at 312 million yuan RMB, according for 5.2% of its total agricultural output values.It can be prophesied that titanium materials will be used increasing in aerospace and inother industries.某种与其他疾病伴发的高血压,称为继发性高血压本节内容如有更改,均见本书末附录。
That a microganismis capable of destroyingone of anther species was first discoveredbyPasteur, who pointed out that can be used to the therapeutic use.It’s truly amazing that they can produce cells that look like embryoric stem cells.It’s no accident of history that the first Earth Day in April 1970 came so soon after thecolor photograghs of the whole earth from space were made by homesick astronauts on theApollo 8 mission to the moon December 1968.There has been no doubt in my mind of the progress which you have achieved.I was all the more delighted when as a result of the initiative of your government,it proved possible to reinstate the visit so quickly.One cannot properly investigate the incredibly complex problems thrown up by the modern world and by recent development in our knowledge along the narrow front of a single discipline.The tiny tropical fish has long interested scientists because of its unique ability to repair damaged and diseased cells in their own eyes.One can never succeed without making great efforts.He felt greatly excited at the thought of going abroad for further study.I would appreciated if samples or brochure could be soon forward to us.I would appreciated it if you can reply as soon as possible.He had flown yesterday from Beijing where he spent his vocation after finishing the meeting he had taken part in Tianjing.为保证最大的分辨率必须选用薄层,为使误差最小必须选用厚层,实际上要权衡一下来选择厚度。
阳极氧化和超疏水协同效应
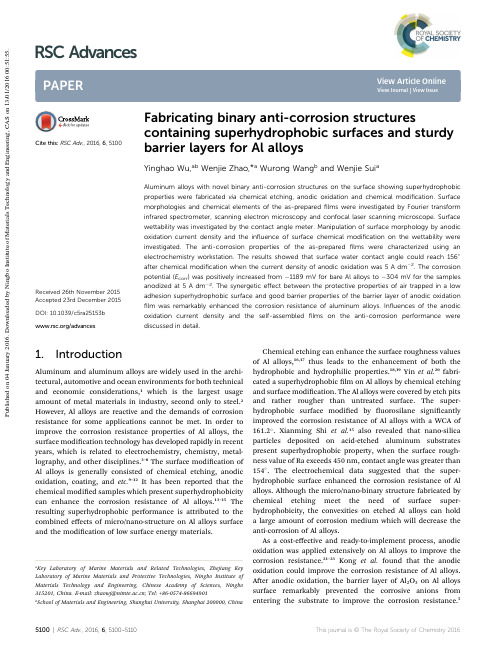
5100 | RSC Adv., 2016, 6, 5100–5110
This journal is © The Royal Society of Chemistry 2016
View Article Online
Paper
RSC Advances
However, there are many nanopores on the anodized sample surfaces, of which the chloride ions in liquid can stay in the pores. As time going on, the chloride ions could permeate the barrier lm and corrode the aluminum substrate. The superhydrophobic surface can protect the aluminum substrate from corrosion.24–26 Li et al.24 fabricated a superhydrophobic surface by anodic oxidation and self-assembly. The inuence of anodic oxidation current density and the self-assembly time were studied. The result of electrochemical measurement indicated that the anti-corrosion performance of Al alloys was greatly improved by the self-assembled superhydrophobic lm. It also showed that changing the anodic oxidation condition can manipulate the surface morphology of Al alloys.27–29 Ludmila Boinovich revealed the synergetic effect of superhydrophobic surface and good barrier properties of the oxide sublayer which fabricated by laser treatment could remarkably enhanced the corrosion resistance of aluminum alloys.30 To the best of our knowledge, many studies have been previously carried out about the independent effect of anodic oxide lms and surface superhydrophobicity on the anticorrosion properties. However, utilizing the synergistic effect of anodic oxide lms and surface superhydrophobicity (especially the regular binary structure fabricated by anodic oxidation) to prepare the anti-corrosion lms, was rarely referred to. In particular, it is necessary to explore the relationship between the anti-corrosion properties and the binary structures on Al alloys surface manipulated by anodic oxidation current density. Inspired by the above-mentioned inuences of surface morphology and chemical modication on corrosion resistance, in this work, Al alloys surfaces were treated by an acid etching, anodic oxidation and chemical modication method. A versatile procedure combining micro/nano-binary structures, anodic oxidation porous lms and further modication with low-surface-energy molecules were proposed to fabricate excellent anti-corrosion lms on Al alloys substrates. The various anodic current densities were used to manipulate the surfaces morphology of Al alloys. Electrochemical and surface analysis methods were used in this paper to revel the corresponding synergetic corrosion resistance of anodic oxide lms with various micro/nano-structure manipulated by anodic oxidation current density and chemical modication.
科技英语翻译
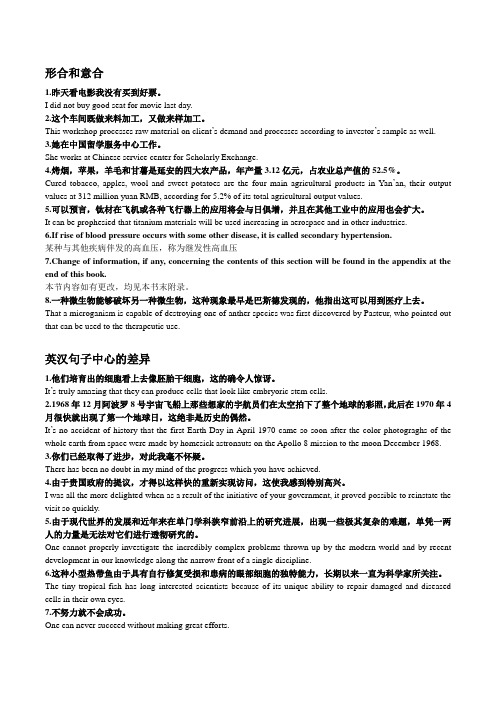
形合和意合1.昨天看电影我没有买到好票。
I did not buy good seat for movie last day.2.这个车间既做来料加工,又做来样加工。
This workshop processes raw material on client’s demand and processes according to investor’s sample as well. 3.她在中国留学服务中心工作。
She works at Chinese service center for Scholarly Exchange.4.烤烟,苹果,羊毛和甘薯是延安的四大农产品,年产量3.12亿元,占农业总产值的52.5%。
Cured tobacco, apples, wool and sweet potatoes are the four main agricultural products in Yan’an, their output values at 312 million yuan RMB, according for 5.2% of its total agricultural output values.5.可以预言,钛材在飞机或各种飞行器上的应用将会与日俱增,并且在其他工业中的应用也会扩大。
It can be prophesied that titanium materials will be used increasing in aerospace and in other industries.6.If rise of blood pressure occurs with some other disease, it is called secondary hypertension.某种与其他疾病伴发的高血压,称为继发性高血压7.Change of information, if any, concerning the contents of this section will be found in the appendix at the end of this book.本节内容如有更改,均见本书末附录。
nanomaterials纳米材料双语
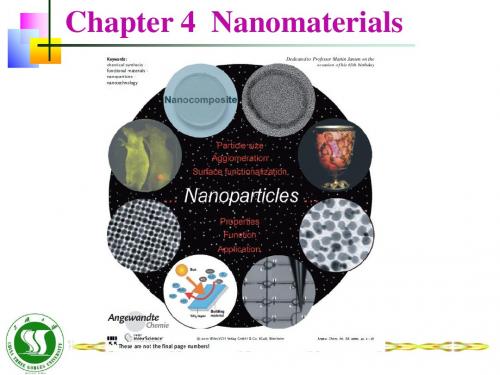
that is sparing of natural resources.
the importance of nanoparticles is due to their
fundamentally novel properties and functions.
I see you, Nemo!
flower NiO
bird’s nest ZnO
Sunflower-Polymer crystal structure
聚对苯二甲酸丙二醇酯(PTT)中添加新型增韧剂(聚丙烯(PP)改性 马来酸酐接枝的乙丙橡胶(EPDM-g-MA))通过双螺杆挤出制得的 样品在偏光显微镜下观察到的晶体形态
4. Natural nanomaterials
Viral capsid
Close-up of the underside of a gecko's foot as it walks on a glass wall. (spatula: 200 × 10-15 nm). "Lotus effect", hydrophobic effect with self-cleaning ability
1. What are Nanomaterials?
describe, in principle, materials of which a single unit is sized (in at least one dimension) between 1 and 1000
nanometers (10−9 metesual
as a quantum size effect.
共价有机框架(COFs)在锂离子电池中的应用
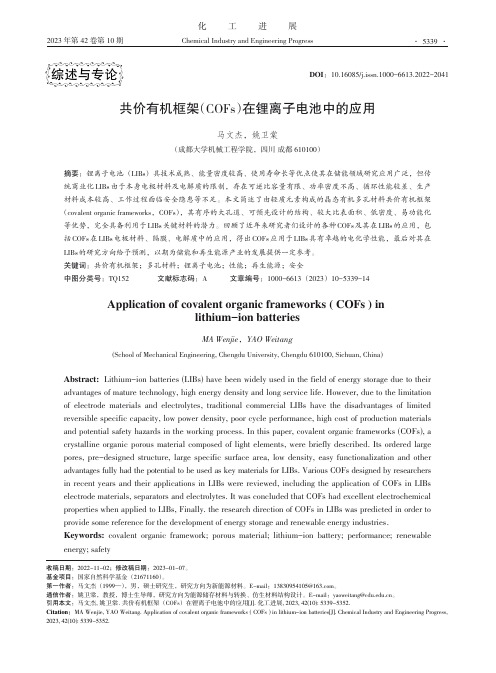
化工进展Chemical Industry and Engineering Progress2023 年第 42 卷第 10 期共价有机框架(COFs )在锂离子电池中的应用马文杰,姚卫棠(成都大学机械工程学院,四川 成都 610100)摘要:锂离子电池(LIBs )具技术成熟、能量密度较高、使用寿命长等优点使其在储能领域研究应用广泛,但传统商业化LIBs 由于本身电极材料及电解质的限制,存在可逆比容量有限、功率密度不高、循环性能较差、生产材料成本较高、工作过程面临安全隐患等不足。
本文简述了由轻质元素构成的晶态有机多孔材料共价有机框架(covalent organic frameworks ,COFs ),其有序的大孔道、可预先设计的结构、较大比表面积、低密度、易功能化等优势,完全具备利用于LIBs 关键材料的潜力。
回顾了近年来研究者们设计的各种COFs 及其在LIBs 的应用,包括COFs 在LIBs 电极材料、隔膜、电解质中的应用,得出COFs 应用于LIBs 具有卓越的电化学性能,最后对其在LIBs 的研究方向给予预测,以期为储能和再生能源产业的发展提供一定参考。
关键词:共价有机框架;多孔材料;锂离子电池;性能;再生能源;安全中图分类号:TQ152 文献标志码:A 文章编号:1000-6613(2023)10-5339-14Application of covalent organic frameworks ( COFs ) inlithium-ion batteriesMA Wenjie ,YAO Weitang(School of Mechanical Engineering, Chengdu University, Chengdu 610100, Sichuan, China)Abstract: Lithium-ion batteries (LIBs) have been widely used in the field of energy storage due to their advantages of mature technology, high energy density and long service life. However, due to the limitation of electrode materials and electrolytes, traditional commercial LIBs have the disadvantages of limited reversible specific capacity, low power density, poor cycle performance, high cost of production materials and potential safety hazards in the working process. In this paper, covalent organic frameworks (COFs), a crystalline organic porous material composed of light elements, were briefly described. Its ordered large pores, pre-designed structure, large specific surface area, low density, easy functionalization and other advantages fully had the potential to be used as key materials for LIBs. Various COFs designed by researchers in recent years and their applications in LIBs were reviewed, including the application of COFs in LIBs electrode materials, separators and electrolytes. It was concluded that COFs had excellent electrochemical properties when applied to LIBs, Finally. the research direction of COFs in LIBs was predicted in order to provide some reference for the development of energy storage and renewable energy industries.Keywords: covalent organic framework; porous material; lithium-ion battery; performance; renewable energy; safety综述与专论DOI :10.16085/j.issn.1000-6613.2022-2041收稿日期:2022-11-02;修改稿日期:2023-01-07。
氨化合成一维GaN纳米线
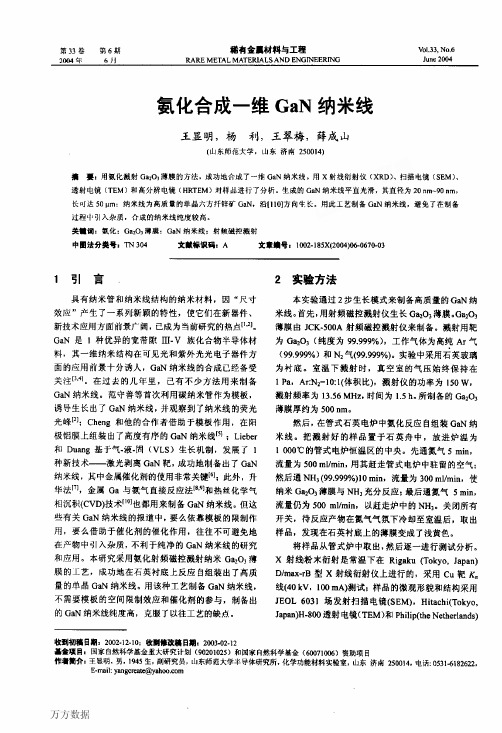
(71):349
【10】Pe“g Y u,zhou T X,Wang N引d,.Bulk.qu蚰tity G州 N蛐oWi增s S”thcsizcd舶m Hot Filament Chemical vapOr
Deposition们.凸Ⅲ聊,如垃2000,(327):263
【11】Chen chi们hun“以CatalyIic GrowⅡl蚰d Char¨Ie一强Hon ofGaIlium Ni仃ide Nanowifes【J】’J一所C冉删SDc,200I,123:
生成了少量的GaN,为氨化时GaN的生长提供了成核 体结构和生长方向与chen等人用催化生长方法制备
结点.有助于GaN纳米线的生长。图1b是l 000℃条 的GiN纳米线相似…l。
件下在NH3气氛中氨化10min后样品的xRD图谱。
图中的衍射峰分别对应着六方晶系GaN相应衍射面
的密勒指数,说明氨化反应生成的浅黄色薄膜为六方
【8】HeM,Minus T’zhou P Z,Mohammed sN剖耐.Growth of Large-scale GaN N蚰owi怫s and Tubes by D{r∞t ReactiOn Of
GawithNHl【J1,』即,.P咖£帆2000,77(23):3 73l
【9】“J Y,chenxL,Qiaoz Y'caoYGHcM,XuL S”thesis of
高,方法简单,避免了由模板限制或催化剂引入的污
(122):188
染,这对于制造纳米尺度的光电子器件和一维GaN纳
[7】Li Y J,Qiao z Y,chen L X甜口,.Morph0109ies of GaN
米材料的基础研究具有十分重要的意义。
onc—dimensionalMatcriaIs【J】一印,取芦』.2000,(71):587
聚变堆第一壁用纳米结构ODS钢的发展与前瞻_吕铮
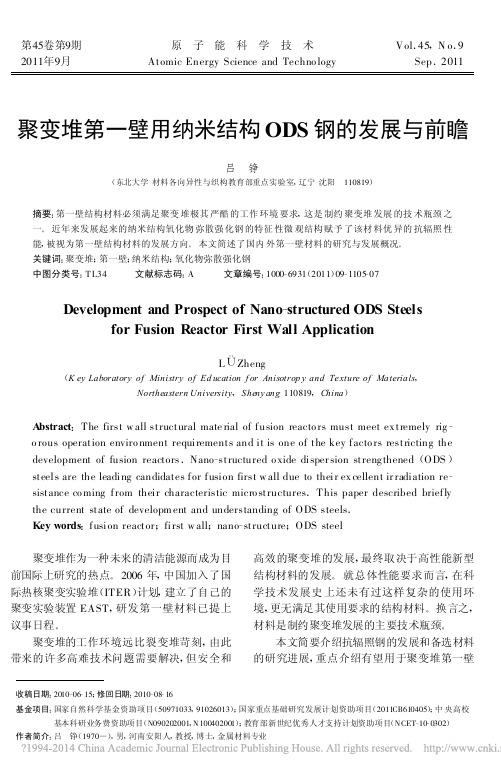
第45卷第9期原子能科学技术Vol .45,N o .9 2011年9月Atomic Energy Science and Techno logy Sep .2011聚变堆第一壁用纳米结构ODS 钢的发展与前瞻吕 铮(东北大学材料各向异性与织构教育部重点实验室,辽宁沈阳 110819)摘要:第一壁结构材料必须满足聚变堆极其严酷的工作环境要求,这是制约聚变堆发展的技术瓶颈之一。
近年来发展起来的纳米结构氧化物弥散强化钢的特征性微观结构赋予了该材料优异的抗辐照性能,被视为第一壁结构材料的发展方向。
本文简述了国内外第一壁材料的研究与发展概况。
关键词:聚变堆;第一壁;纳米结构;氧化物弥散强化钢中图分类号:T L34 文献标志码:A 文章编号:1000-6931(2011)09-1105-07Development and Prospect of Nano -structured ODS Steelsfor Fusion Reactor First Wall ApplicationL Zheng(K ey Laboratory of Ministry of Ed ucation f or Anisotrop y and Te xture of Materials ,收稿日期:2010-06-15;修回日期:2010-08-16基金项目:国家自然科学基金资助项目(50971033,91026013);国家重点基础研究发展计划资助项目(2011CB610405);中央高校基本科研业务费资助项目(N090202001,N100402001);教育部新世纪优秀人才支持计划资助项目(NCET -10-0302)作者简介:吕 铮(1970—),男,河南安阳人,教授,博士,金属材料专业Northeastern University ,Sheny ang 110819,China )A bstract :The first w all structural mate rial of fusion reacto rs must meet ex tremely rig -o rous operation enviro nment requirements and it is one of the key facto rs restricting the development of fusion reactors .Nano -structured o xide disper sion streng thened (ODS )steels are the leading candidates for fusion first w all due to their ex cellent ir radiation re -sistance co ming from their characteristic micro structures .This paper described briefly the current state of developm ent and understanding of ODS steels .Key words :fusion reactor ;first w all ;nano -structure ;ODS steel 聚变堆作为一种未来的清洁能源而成为目前国际上研究的热点。
纳米材料专业英语词汇

纳米尺度nanoscale纳米基元nano-unit纳米结构单元nanostructure unit纳米材料nanomaterial纳米技术nanotechnology纳米结构体系nanostructure system纳米组装体系nanostructure assembling syst em纳米器件nanodevice碳纳米管carbon nanotubes原子团簇atom cluster单分散颗粒[系] monodispersed particle纳米颗粒nanoparticle团粒aggregate纳米粉体nano-powder纳米纤维nano-fibre纳米薄膜nano-film纳米块体nano-bulk纳米孔nano-pore纳米晶体材料nanocrystalline material纳米非晶材料amorphous nanomaterial纳米准晶材料quasi-crystal nanomaterial金属纳米材料metallic nanomaterial无机非金属纳米材料inorganic non-metallic na nomaterial高分子纳米材料polymer nanomaterial纳米复合材料nanocomposites结构纳米材料structured nanomaterial功能纳米材料functional nanomaterial生物医用纳米材料biomedical nanomaterial 小尺寸效应small-size effect表面效应surface effect量子尺寸效应quantum size effect宏观量子隧道效应macroscopic quantum tun neling effect惰性气体沉积法inert gas deposition物理粉碎法physics grinding高能球磨法high energy ball mill溅射法sputtering物理粉碎法physics grinding爆炸法explosion喷雾法spraying冷冻干燥法freeze drying化学气相沉积法chemical vapor deposition沉淀法precipitation水热合成法hydrothermal synthesis溶胶-凝胶法sol-gel辐射化学合成法radiation chemical synthesis 快速凝固法rapidly quenching强烈塑性变形法severe(intense) plastic deform ationh非晶晶化法amorphous solid crystallizatio n溅射法sputtering非晶晶化法crystallization of amorphous soli d原位复合法in-situ composite插层复合法intercalation hybrids微乳液法micro emulsion模板合成法template synthesis自组装法self-assembly石墨电弧放电法graphite arc discharge快速凝固法rapidly quenching表面处理surface treatment表面修饰surface decoration稳定化处理passivating treatmentX射线衍射法X-ray diffractometry扫描探针显微镜scanning probe microscopy 扫描隧道显微镜scanning tunneling microscop y,扫描近场光学显微镜scanning near-field optica l microscopy,原子力显微镜atomic force microscopy扫描电容显微镜scanning capacitance microsc opy磁力显微镜magnetic force microscopy扫描热显微镜scanning thermal microscopy X射线衍射法X-ray diffractometryX射线衍射线宽化法X-ray diffractometry line b roadeningX射线小角度散射法small angle X-ray scatteri ng透射电子显微镜法transmission electron micro scopy ,TEM透射电镜法TEM method扫描电子显微镜法scanning electron microsco py , SEM扫描电镜法SEM method拉曼光谱法raman spectrometry红外吸收光谱法infrared absorption spectrosc opy穆斯堡尔谱法mossbauer spectrometry光子相关谱法photon correlation spectroscop yBET法BET压汞仪法mercury porosimetry纳米压痕仪nano impress扫描探针显微法scanning probe microscopy, 扫描隧道电子显微法scanning tunneling electr on microscopy,STM扫描近场光学显微法scanning near-field optica l microscopy,SNOM原子力显微法atomic force microscopy,AFM 扫描电容显微法scanning capacitance micros copy, SCM扫描热显微法scanning thermal microscopy, STHM场离子显微法field ion microscopy, FIM磁力显微法magnetic force microscopy, MFM 激光干涉仪laser interferometer激光衍射/散射法laser diffraction and scatterin g离心沉降法centrifugal sedimentation。
纳米粒子合成方法综述Controlled_synthesis_of_semiconductor_nanostructures_in_the_liquid_phase
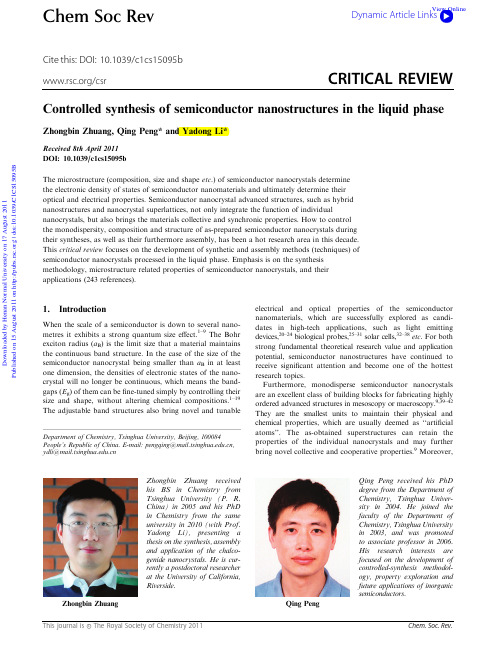
Cite this:DOI:10.1039/c1cs15095b Controlled synthesis of semiconductor nanostructures in the liquid phaseZhongbin Zhuang,Qing Peng*and Yadong Li*Received 8th April 2011DOI:10.1039/c1cs15095bThe microstructure (composition,size and shape etc.)of semiconductor nanocrystals determine the electronic density of states of semiconductor nanomaterials and ultimately determine their optical and electrical properties.Semiconductor nanocrystal advanced structures,such as hybrid nanostructures and nanocrystal superlattices,not only integrate the function of individualnanocrystals,but also brings the materials collective and synchronic properties.How to control the monodispersity,composition and structure of as-prepared semiconductor nanocrystals during their syntheses,as well as their furthermore assembly,has been a hot research area in this decade.This critical review focuses on the development of synthetic and assembly methods (techniques)of semiconductor nanocrystals processed in the liquid phase.Emphasis is on the synthesis methodology,microstructure related properties of semiconductor nanocrystals,and their applications (243references).1.IntroductionWhen the scale of a semiconductor is down to several nano-metres it exhibits a strong quantum size effect.1–9The Bohr exciton radius (a B )is the limit size that a material maintains the continuous band structure.In the case of the size of the semiconductor nanocrystal being smaller than a B in at least one dimension,the densities of electronic states of the nano-crystal will no longer be continuous,which means the band-gaps (E g )of them can be fine-tuned simply by controlling their size and shape,without altering chemical compositions.1–19The adjustable band structures also bring novel and tunableelectrical and optical properties of the semiconductor nanomaterials,which are successfully explored as candi-dates in high-tech applications,such as light emitting devices,20–24biological probes,25–31solar cells,32–38etc.For both strong fundamental theoretical research value and application potential,semiconductor nanostructures have continued to receive significant attention and become one of the hottest research topics.Furthermore,monodisperse semiconductor nanocrystals are an excellent class of building blocks for fabricating highly ordered advanced structures in mesoscopy or macroscopy.9,39–42They are the smallest units to maintain their physical and chemical properties,which are usually deemed as ‘‘artificial atoms’’.The as-obtained superstructures can retain the properties of the individual nanocrystals and may further bring novel collective and cooperative properties.9Moreover,Department of Chemistry,Tsinghua University,Beijing,100084People’s Republic of China.E-mail:pengqing@,ydli@Zhongbin ZhuangZhongbin Zhuang received his BS in Chemistry from Tsinghua University (P.R.China)in 2005and his PhD in Chemistry from the same university in 2010(with Prof.Yadong Li),presenting a thesis on the synthesis,assembly and application of the chalco-genide nanocrystals.He is cur-rently a postdoctoral researcher at the University of California,Riverside.Qing PengQing Peng received his PhD degree from the Department of Chemistry,Tsinghua Univer-sity in 2004.He joined the faculty of the Department of Chemistry,Tsinghua University in 2003,and was promoted to associate professor in 2006.His research interests are focused on the development of controlled-synthesis methodol-ogy,property exploration and future applications of inorganic semiconductors.Chem Soc RevDynamic Article Links/csrCRITICAL REVIEWD o w n l o a d e d b y H e n a n N o r m a l U n i v e r s i t y o n 17 A u g u s t 2011P u b l i s h e d o n 15 A u g u s t 2011 o n h t t p ://p u b s .r s c .o r g | d o i :10.1039/C 1C S 15095BView Onlinethrough controlling the structure and packing of the building-block nanocrystals,it also gives the possibility to construct desired nanocrystal superlattices and tailor their properties.42This ‘‘bottom–up’’assembly provides an alternative way to integrate and improve the performance of nanomaterials,and may have potential applications for the development of future nanodevices.In order to achieve the specific properties not only for semiconductor nanocrystals,but also for the successive nano-crystal superlattices,development of synthetic methods are required to control the monodispersity,composition and structure (size and shape,or other parameters)of the as-prepared building-block semiconductor nanocrystals during their syntheses.A successful synthesis is usually the basis of their further applications.New synthetic methods will allow us to prepare nanomaterials with novel structures,which will bring new properties.In recent years,some well-defined and effective synthetic methods have been developed and thorough researches,correlated with controlled synthesis of semiconductor nanostructures,have been carried out by material scientists all over the world.Although the precise control of nanocrystals (such as the desired size,shape,exposed facets)is still a challenge,various methods with good commonality and fine controllability have been reported.16–18,43–49The related researches are also helpful to understand the growth mechanism of nanocrystals and the structurally dependent chemical and physical properties at the microscopic level.In this review,we summarize the synthetic methods for semiconductor nanocrystals and their superlattices in the liquid phase.We also attempt to find general rules in reaction system design and expect then to guide the material synthesis.The semiconductor compounds discussed in this review are limited to metal compounds with VIA and VA elements (the II–VI and III–V semiconductors),comprising the sulfides,selenides,tellurides,as well as the nitrides,phosphides,arsenides.Metal oxides are excluded and several excellent reviews have discussed them in detail.16,18,45,50–53Elemental solid-state S,Se,Te and P,As have relatively low reactivities,which makes the traditional preparation method of these semiconductors limited to the direct combination of the elements at high temperature (usually at B 10001C).54–57Molecular beam epitaxy (MBE),58–64metal–organic vapor chemical deposition(MOCVD),58,65–68and organometallic vapor phase epitaxy (OMVPE)69–75methods have also been adopted for preparing films and coatings.However,semiconductor nanocrystals are always metastable phases,and they should be passivated by ligands to avoid aggregation,though it is hard to meet this goal by the above mentioned solid–solid or solid–gas reactions.Synthetic methods through liquid-phase processes have shown powerful controllability in the preparation of semiconductor nanostructures.In a liquid reaction,it is very easy to introduce various ligands to passivate the nanocrystals and meanwhile adjust their nucleation and growth.The chemical potentials of the reactants can be easily adjusted and the reaction temperature is usually below 3001C.Furthermore,additional controlling techniques can also be carried out conveniently,such as confining the reaction at the interface,making small reaction chambers or using phase separation to slowly release the reactant.Obviously,reaction in the liquid phase has been adopted as the most favorable synthetic method for semi-conductor nanocrystals.Such reactions are emphasised in this review.In this review,first,we describe the basic theory on crystalli-zation from solution.These general theories may guide us to choose the reactants and design the reaction systems.Then five kinds of reaction systems are discussed in detail:chemical precipitation,thermal decomposition,solvothermal,hydrothermal and interface controlled reaction systems.We lay emphases on the design of the reaction system and the choice of the reactants.We also discuss the features and mechanism of the reactions and try to understand rules in the reaction systems.Finally,we discuss assembly building by the as-obtained nanocrystals,which is a ‘‘bottom–up’’approach to integrate nanocrystals and further fabricate devices.2.General theory on crystallization in the liquid phaseIn the liquid-phase synthesis of semiconductor nanocrystals,the precursors are dispersed in the solvent and the products are the new-phase species generated from the solution.The synthetic process can be simply recognized as the chemical reaction between the precursors to produce monomers,and then the monomers aggregate into crystals.Thus the nucleation and growth of the particles are the two key points during the controlled synthesis.Generally,the nucleation process can be classified into two types,homogenous and heterogeneous nucleation.Homo-genous nucleation occurs when the reaction system does not have a solid–liquid interface to help the Mer and co-workers 76have discussed the separation of nucleation and growth in 1950and provided a theoretical model.The nuclei are a new phase generated from the solution since the oversaturated solution is unstable.The free energy change (D G )of the nucleation process can be described as follows (eqn (1),assuming that the nuclei are spherical):D G ¼À4p r 3k B T ln ðS Þþ4p r 2g ð1Þwhere V is the volume and r is the radius of the nuclei,k B is the Boltzmann constant,S is the saturation ratio,and g is theunitYadong LiYadong Li received his PhD degree in Chemistry from the University of Science and Technology of China in 1998.He joined the faculty of the Department of Chemistry,Tsinghua University,in 1999as a full professor.His research interests are focused on synthesis,assembly,struc-ture and application explora-tion of nanomaterials.D o w n l o a d e d b y H e n a n N o r m a l U n i v e r s i t y o n 17 A u g u s t 2011P u b l i s h e d o n 15 A u g u s t 2011 o n h t t p ://p u b s .r s c .o r g | d o i :10.1039/C 1C S 15095Bsurface free energy.The overall free energy change is the sum of two terms.The first term comes from the formation of new volume from the solution and the second part comes from the new surface generated.When the S is greater than 1,the volume part is negative and the system is tending to precipitate solid species.Thus higher S is beneficial for the nucleation.However,the newly generated surface has positive energy and interrupts the formation of the nuclei.There is a critical r (r *)for a given value of S ,and it can be calculated by setting d D G /d r =0,eqn (2).r ü2V g 3k B T ln ðS Þð2ÞWhen the nuclei are larger than the critical size,they will further decrease their free energy by growth and form stable nuclei that grow to form particles.Furthermore,from the above equation,it is known that the r *is related to S ,and larger S gives smaller r *.This means that a high saturation ratio is of benefit to the nucleation from solution.The heterogeneous nucleation needs an additional solid surface to help the crystallization and the monomers will epitaxially grow on the pre-established nuclei.Thus apart from the influence of S ,the mismatch of the cell structure is also an important factor.77–81The methods using assistance of seeds usually show this type of nucleation.With monomers continuously precipitating on the nuclei the growth process of the nanocrystals would occur.Due to the anisotropy of the crystal,the energies needed in the growth of nanocrystals along different directions are not the same.Nanocrystals grow along the favorable directions and result in special morphologies.By adjusting the reaction conditions,as to whether anisotropy to nanocrystals is expressed,the growth of the nanocrystal could be controlled.Apart from the intrinsic difference in the crystal structures,the surface energy can also be adjusted on the selected facets by using specific binding molecules.The absorption of the foreign molecule on the surfaces of nuclei is quite different when the faces are different.82–90Thus the growth of the nanocrystals can be also controlled by the additional ligands.In order to control the synthesis of nanostructures,a rational reaction system should be designed.First,the selection of a appropriate precursor is very critical for the reaction.The rate of the monomer generation is related to the reactivity of the precursors,which is effective on the saturation ratio,and will further influence the nucleation and growth.Secondly,a reaction system with rational conditions is also helpful to control the growth of the nanocrystals.The reaction condi-tions (such as the concentration,temperature,pH etc.)are related to the chemical potentials of the reactants,which are important factors to the stability of nuclei and nano-crystals.Thirdly,the surface energies can be adjusted by additional ligands,which can make the nanocrystals grow along favorable directions and precisely control the morphology of the as-obtained nanocrystals.Next,we will introduce five main kinds of reaction systems in the liquid phase,which are effective approaches to synthe-size semiconductor nanocrystals.3.Chemical precipitationAlmost three decades ago,the effect of size on optical properties of semiconductor nanocrystal materials was discovered.Ekimov and co-workers 91,92performed a remarkable study of quantum confinement effects on semiconductor nano-particles embedded in glass while Brus and co-workers had done the pioneering work on colloidal nanoparticles.93–95The basic purpose was to explore their size-dependent effects in the excited electronic states,which raised the interest of materials in nanoscale size and with adjustable diameters.The early works were mainly focused on fluorescent nanocrystals,especially on CdS and ZnS.94,96,97It was shown that quantum size effects were clearly influencing the optical properties of these particles prepared in liquid solution.The synthetic methods were always basic chemical precipitation based on traditional colloidal chemistry,where metal cations directly react with inorganic chalcogenide sources,e.g.eqn (3).M n þþS 2À !aqueous solutionM n =2Sð3ÞBased on the successful synthesis of these nanoparticles,Brus et al.worked out the basic scaling laws that describe the evolution of the optical spectra vs .size.The relationship is shown in eqn (4):D E ffi h 2p 22R 1m e þ1m Ãh À1:8e 2e R ð4Þwhere R represents the radius of nanocrystals,e the elementarycharge,e the bulk optical dielectric coefficient,m e *and m h *the effective mass of the electron and hole,respectively.The size of nanoparticles is found to strongly influence the band gap of semiconductors at the nanoscale.To control the size of nanocrystals,Henglein and co-workers 98prepared CdS nanoparticles with strong luminescence by reacting Cd 2+with H 2S under carefully controlled pH.Weller and co-workers 99developed this method to obtain a more complex CdS/HgS/CdS core/shell structure by a multiple precipitation process.During the precipitation,additional foreign stabilizing molecules can be introduced to the system to avoid the further growth and aggregation of the nano-crystals (by absorbing on the surface of the nanocrystals).Brus and co-workers 100synthesized CdS nanocrystals by the reaction of CdSO 4and (NH 4)2S.Styrene/maleic anhydride copolymer is used as stabilizing reagent.The size of the nanocrystals can be varied from 2.1to 7.5nm.Another method to control the growth of semiconductor nanocrystals is using inverse micelles.The inverse micelle has nanometre sized water droplets,which provides a chamber where the precipitation reaction occurs and also confines the further growth of particles.Alivisatos and co-workers 101synthesized CdS nanocrystals by limiting the reaction of Cd(ClO 4)2and Na 2S in the water droplets of the micelle formed by dioctyl sulfosuccinate (AOT).Similarly,Stroeve and co-workers 102synthesized ZnSe nanocrystals by using Zn(ClO 4)2and Na 2Se in the same AOT micelle system (eqn (5)).M ðClO 4Þ2þNa 2S =Na 2Se !H 2O Àheptane ÀAOTMS =MSeð5ÞD o w n l o a d e d b y H e n a n N o r m a l U n i v e r s i t y o n 17 A u g u s t 2011P u b l i s h e d o n 15 A u g u s t 2011 o n h t t p ://p u b s .r s c .o r g | d o i :10.1039/C 1C S 15095BAlthough these pioneering works have successfully synthesizedsome semiconductor materials with nanometre size,however,the direct combination of free inorganic ions in aqueous solution makes the precipitation rate too fast to control.The final size and shape of the as-obtained semiconductor nano-crystals is usually not uniform.The reaction temperature is also relatively low and thus the as-obtained nanocrystals generally have poor crystallinity.The above earlier works have shown that many special phenomena occur when the size of semiconductors is down to the nano-scale.A basic model has been established to investigate the unique properties of these nanomaterials.The basic relationship between the size and band structures has been studied by both experiment and theory,and sub-sequently,research of semiconductor nanocrystals has become a hot topic.To further explore the structure related properties of nanocrystals and achieve the potential of their applications,synthetic methods to prepare high-quality semiconductor nanocrystals with more controllability were urgently needed and this promoted the development of the synthetic metho-dology for semiconductor nanomaterials.4.Thermal decompositionThe thermal decomposition method employs organometallic compounds containing metals and chalcogenides as the pre-cursors,which decompose at relatively high temperature in a high boiling-point organic solvent.High-quality nanocrystals with narrow size distributions and good crystallinity could be produced.The key point to achieve monodisperse nano-crystals is the separation of nucleation and growth of thenanocrystals during their syntheses.It needs a short,fast nucleation period at the beginning and then the nuclei grow without further nucleation.To reach this goal,generally,hot-injection technology is employed to achieve the burst nucleation in the system by rapidly mixing two highly reactive precursors.By carefully choosing appropriate precursors,some non-injection methods are also developed successfully.In addition,by using pre-existing seeds as the nuclei to induce the nanocrystals growth,nanowires with unique diameters could be obtained,which is known as the solution-liquid–solid (SLS)mechanism.4.1Hot-injection based synthesisIn order to produce a large amount of monomers during a short term to trigger a burst nucleation in the reaction system,the hot-injection technique,which consists of rapidly mixing highly reactive precursors at a certain elevated temperature,was established.In 1993,Bawendi and co-workers 103reported the first synthesis of high-quality CdSe nanocrystals by mixing Cd(CH 3)2and TOPSe in TOPO (a high boiling,weakly polar and coordinating solvent)at 230–2601C (eqn (6)).Cd ðCH 3Þ2þTOPSe !TOPO ;230À260 CCdSeð6ÞFrom then on,this method has been successfully adopted and widely used to synthesize other semiconductor nanocrystals,such as CdS,103CdTe,103ZnSe,104–106ZnS,106PbS,107PbSe,108,109PbTe,110,111InAs,112,113InP 113,114etc.Generally,during the hot-injection based synthesis,there are usually two approaches to control the growth of nano-crystals.The first is choosing different ligands to adjust the surface energy (by absorbing on the surface of the nanocrystal)of a certain facet of nanocrystals,which may direct the nanocrystals growing along a favorable direction.Alivisatos and co-workers 115have synthesized rod-like CdSe nano-crystals in the reaction system with a high concentration of hexylphosphonic acid (HPA).The other is to adjust the chemical potential of the monomer in the reaction system,which is usually done by controlling the concentration of reactants.For nanocrystals with anisotropic crystal structures,such as wurtzite CdSe,by increasing the concentration of monomer,the intrinsic anisotropic feature of crystal structure may be magnified,which leads to the orientational growth of nanocrystals.Peng and co-workers 83,84reported the syntheses of dot-like,rod-like and tetrapod wurtzite CdSe nanocrystals under different monomer concentrations (Fig.1).Further studies show that greener precursors with low activity (such as CdO,instead of Cd(CH 3)2)and non-coordinating solvents (such as 1-octadecene,ODE)can also be used in modified hot-injection based syntheses.116–118For more information,please refer to some specialized reviews correlated with hot-injection based synthesis of II–VI semiconductor nanocrystals.9,17,43,44,119,120Similarly,III–V semiconductor nanocrystals can also be synthesized through this hot injection method.Typically,TMS 3P and TMS 3As are selected as the group V element sources and they are injected into a hot coordinating solvent containing the In or Ga source,which are usuallycarboxylateFig.1TEM images of CdSe nanocrystals with various morphologies obtained by the hot-injection based thermal decomposition approach:(a)monodisperse dots;(b)nanorods;(c)rice-shaped nanocrystals;(d)tetrapods (reproduced with permission from ref.83,copyright 2001,American Chemical Society,and ref.84,copyright 2002,American Chemical Society).D o w n l o a d e d b y H e n a n N o r m a l U n i v e r s i t y o n 17 A u g u s t 2011P u b l i s h e d o n 15 A u g u s t 2011 o n h t t p ://p u b s .r s c .o r g | d o i :10.1039/C 1C S 15095Bsalts.The pioneering work was carried out by Nozik and co-workers in 1994to synthesis InP quantum dots,eqn (7).114Chloroindium oxalate þTMS 3P !TOPO ;270 CInPð7ÞIn recent years Peng and co-workers 113have synthesized high-quality InP and InAs nanocrystals in non-coordinating solvents by using fatty acids as ligands.Banin and co-workers 112have synthesized InAs/InP and InAs/CdSe core/shell structure nanocrystals using TOP as ligands through a two-step routine.Through the hot-injection method,high-quality semi-conductor nanocrystals can be obtained without further size separation.They have narrow size distributions and show good monochromaticity of photoluminescence,which can be used for electrical devices,such as light emitting devices (LEDs).The nanocrystal based LEDs have pure and saturated emission colors with narrow bandwidth,and their emission wavelength can be easily tuned by changing the size of the nanocrystals.Colvin and co-workers 20prepared quantum dot LEDs by using CdSe nanocrystals as the emitting and electron transporting layer and a semiconducting polymer (p -para-phenylene vinylene).This first quantum dot LEDs had very low external quantum efficiency,but later Bawendi and co-workers 121improved the construction by putting the nano-crystals between the hole and electron transport organic layer.Recently,Li and co-workers 122further developed the structure and obtained high-performance LEDs;a trilayered structure was adopted and quantum dots (QDs)were used as emissive layers.They found that the thicknesses of semiconductor nanocrystals were quite important for the electroluminescent efficiency.At the optimal thicknesses,they reached the maximum luminance values of 9064,3200,4470,3700cd m À2for red,orange,yellow and green LEDs,respectively.The white LED,which is good candidate in the lighting industry and backlighting applications in displays,can also be achieved by mixing several kinds of semiconductor nanocrystals.For example,Gigli and co-workers 123fabricated bright white LED by using CdSe/ZnS quantum dots with three different sizes.Bawendi and co-workers 124demonstrated a kind of white light LED by electroluminescence from a mixed monolayer of red,green and blue emitting quantum dots.The red quantum dots solution consisted of a CdSe/ZnS core–shell structure;the green quantum dots solution consisted of ZnSe/CdSe alloyed core overcoated with a shell of ZnS,and the blue quantum dots solution consisted of ZnCdS alloyed quantum dots.The white LED were prepared by mixing red,green and blue quantum dots solution with the ratio of 1:2:10for R :G :B.Semiconductor nanocrystals can also be used as biological labels to replace traditional organic dyes.To apply them in the biological applications,the as-obtained nanocrystals should be first transferred into aqueous solution by a surface modification step.Mercaptohydrocarbonic acids were used to transfer the nanocrystals into water.27,125The thiol group anchors on the surface of the nanocrystals and the carboxyl group on the other side makes the nanocrystals water soluble.Some other general approaches have also been developed by using water-soluble ligands or polymers.126,127An alternative approach is growth of a silica shell around the nanocrystals.26The charge of the silica makes the nanocrystal water soluble andit can be further functionalized.Upon modification of the nanocrystals with bio-functional molecules they can be used in biological labeling.In 1998,Alivisatos and co-workers 26reported pioneering work about quantum dot labeling.Water-soluble CdSe@CdS was prepared through coating with a silica layer by employing silanes with various functional groups,such as (3-mercapto-propyl)trimethoxysilane.Then they combined the functional groups with biotin to make a biotinylated nanocrystal.Such modified quantum dots can be used to label fibroblasts,which have been incubated in phalloidin-biotin and streptavidin.At the same time,Nie and co-workers 27developed a class of nonisotopic detection labels by coupling luminescent semicon-ductor quantum dots to biological molecules.CdSe@ZnS quantum dots were adopted and mercaptoacetic acid was used for the solubilization of the nanocrystals in water,as well as for covalent protein or biomolecule attachment.The transfer-ring quantum dot bioconjugates could be used for cultured HeLa cells and immunoglobulin G (IgG)labeling.Subsequently,semiconductor nanocrystals have been adopted as a new class of fluorescent pared to organic dyes,quantum dots are robust and bright light emitters.Along with the research in this decade,more and more work has shown the great potential of semiconductor quantum-dot labeling in biologic fields.29,128,129However,there are still problems,such as the toxicity of cadmium and the metabolism of quantum dots in the body.More bright and non-toxic quantum dots are still desired.4.2Single precursor approachSome single precursor approaches are also developed for the synthesis of semiconductor nanocrystals.This method employs special precursors containing both metal and corresponding chalcogen elements.They can decompose under a certain temperature to generate semiconductor compounds directly.With ligands existing in the reaction system,the size and shape of the semiconductor nanocrystals can be controlled.The key point for the success of this method is the selection of a suitable precursor.Clusters and coordination compounds are favorable choices because the central metal ions and ligands can provide all the atoms that the semiconductor compound require.O’Brien and co-workers 130obtained CdSe nanocrystals by using the precursor [CH 3CdSe 2CN(C 2H 5)2]2decomposing in hot TOPO.Strouse and co-workers 131used [M 10Se 4(SPh)16]4À(where M =Cd,Zn)to synthesize CdSe and ZnSe nanocrystals in hexadecylamine solvent.However,the precursors used for the single precursor method are often hard to synthesise and also limited in type.Korgel and co-workers 132,133developed a solventless method to synthesize Cu 2S nanocrystals by using the metal thiolate as the single precursor,which can be easily obtained by simply mixing Cu salts and thiols.The long chain thiol part in the precursor provides sulfur and also acts as the ligand to control the nanocrystal growth.4.3Solution–liquid–solid (SLS)mechanismThe above mentioned approaches are generally used for the synthesis of zero-dimensional nanocrystals,such as quantumD o w n l o a d e d b y H e n a n N o r m a l U n i v e r s i t y o n 17 A u g u s t 2011P u b l i s h e d o n 15 A u g u s t 2011 o n h t t p ://p u b s .r s c .o r g | d o i :10.1039/C 1C S 15095B。
不同材料表面构筑水凝胶涂层的通用策略
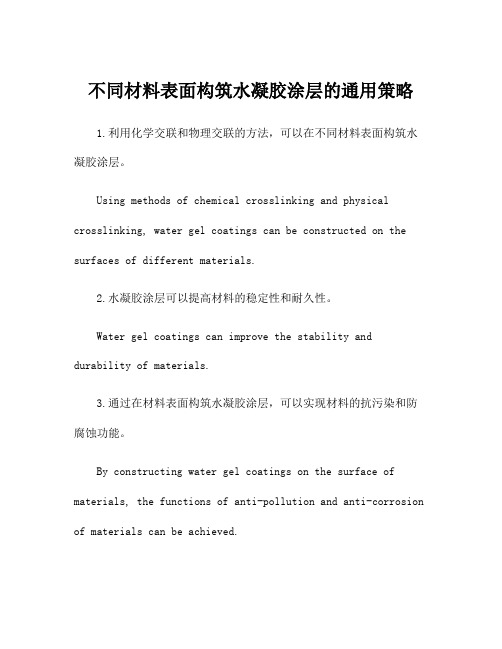
不同材料表面构筑水凝胶涂层的通用策略1.利用化学交联和物理交联的方法,可以在不同材料表面构筑水凝胶涂层。
Using methods of chemical crosslinking and physical crosslinking, water gel coatings can be constructed on the surfaces of different materials.2.水凝胶涂层可以提高材料的稳定性和耐久性。
Water gel coatings can improve the stability and durability of materials.3.通过在材料表面构筑水凝胶涂层,可以实现材料的抗污染和防腐蚀功能。
By constructing water gel coatings on the surface of materials, the functions of anti-pollution and anti-corrosion of materials can be achieved.4.超疏水表面结构可以用于构筑水凝胶涂层,提高材料的防水性能。
Superhydrophobic surface structure can be used to construct water gel coatings to improve the waterproof performance of materials.5.聚合物材料在表面构筑水凝胶涂层可以提高其抗撕裂性能。
Constructing water gel coatings on the surface of polymer materials can improve their tear resistance performance.6.金属材料的耐蚀性能可以通过构筑水凝胶涂层得到显著提升。
The corrosion resistance of metal materials can be significantly improved by constructing water gel coatings.7.纳米材料表面构筑水凝胶涂层可以增强其光电性能和化学稳定性。
- 1、下载文档前请自行甄别文档内容的完整性,平台不提供额外的编辑、内容补充、找答案等附加服务。
- 2、"仅部分预览"的文档,不可在线预览部分如存在完整性等问题,可反馈申请退款(可完整预览的文档不适用该条件!)。
- 3、如文档侵犯您的权益,请联系客服反馈,我们会尽快为您处理(人工客服工作时间:9:00-18:30)。
Journal of Power Sources 196 (2011) 6951–6955Contents lists available at ScienceDirectJournal of PowerSourcesj o u r n a l h o m e p a g e :w w w.e l s e v i e r.c o m /l o c a t e /j p o w s o urShort communicationA nano-structured and highly ordered polypyrrole-sulfur cathode for lithium–sulfur batteriesXiao Liang,Yu Liu,Zhaoyin Wen ∗,Lezhi Huang,Xiuyan Wang,Hao ZhangCAS Key Laboratory of Materials for Energy Conversion,Shanghai Institute of Ceramics,Chinese Academy of Sciences,Shanghai 200050,PR Chinaa r t i c l e i n f o Article history:Received 26August 2010Received in revised form 22November 2010Accepted 23November 2010Available online 30 November 2010Keywords:SulfurTubular polypyrrole Lithium–sulfur battery Composite cathodea b s t r a c tA tubular polypyrrole (T-PPy)fiber is synthesized as a conductive matrix for the cathode of lithium–sulfur secondary battery.The sublimed sulfur is incorporated with the T-PPy by a co-heating process.The loca-tion and the content of sulfur show a significant effect on the electrochemical behavior of the composite.A reversible capacity of ca.650mAh g −1is maintained for over 80cycles for the S/T-PPy composite with 30wt.%sulfur.The enhanced conductivity,the favorable distribution of the nano-sized sulfur in the T-PPy and the stable retention of polysulfides lead to the improvement of the cycling stability of the sulfur based electrode.© 2010 Elsevier B.V. All rights reserved.1.IntroductionRechargeable lithium/sulfur (Li/S)battery shows a high theo-retical capacity of 1675mAh g −1,which is nearly one magnitude higher than that of the currently commercialized LiFePO 4-based Li-ion battery [1–4].Sulfur also has the advantages of low cost,low toxicity and abundance as the cathode material [5].However,poor electrical conductivity of sulfur and intrinsic sulfide shuttle seriously hinder the development of Li/S battery [6,7].The lithium polysulfides generated during the charge/discharge process is sol-uble in organic electrolyte,which leads to the loss of the active material in the cathode [8].Moreover,the soluble polysulfides deposit on the surface of the anode by a shuttle mechanism,which aggravates the corrosion of the anode and increases the internal resistance of the cell,resulting in a rapid fading of the reversible capacity [9].Many efforts have been devoted to improve the cycling stability of the Li/S battery,mainly focusing on the combination of a conductive matrix with sulfur to form a highly conductive composite.Mesoporous carbon,acetylene black and multiwalled carbon tube have been reported as an absorbing and conducting matrix for sulfur electrode due to their highly porous structure and good electronic conductivity [10–12].Polypyrrole is another con-ductive polymer with good compatibility with sulfur and organic electrolytes [13,14].The morphology of polypyrrole represents a key to improve the electrochemical performance of sulfur.In this∗Corresponding author.Tel.:+862152411704;fax:+862152413903.E-mail addresses:yuliu@ (Y.Liu),zywen@ (Z.Wen).study,a tubular polypyrrole (T-PPy)was proposed as the conduc-tive matrix.The S/T-PPy composites were prepared by co-heating the sublimed sulfur with the tubular PPy at a suitable tempera-ture.The electrochemical behaviors of the S/T-PPy composites were investigated.2.ExperimentalThe tubular polypyrrole (T-PPy)was synthesized by a self-degraded template method [15].210ml aqueous solution of FeCl 3(10.5mmol)and methyl orange (1.05mmol)was prepared as the template.10.5mmol pyrrole was added into the template and stirred for 24h.The product was washed by water and ethanol alternatively until the filtrate was colorless.The as-obtained tubu-lar PPy was further dried under vacuum at 50◦C for 12h.Mixtures of sulfur and the tubular PPy in the weight ratio of 1:1and 1.5:1were co-heated at 150◦C for 3h.During the heating process,the sulfur was molten and filled to the holes of the tubular PPy.The mixtures were further heated at 300◦C for 2h to vaporize the sulfur remained on the outside surface of the tubular PPy.Two types of S/T-PPy com-posites with 30wt.%and 50wt.%sulfur were prepared,respectively,as reported in Ref.[13].The processes were performed in Ar atmo-sphere.Phase of the samples was characterized by X-ray diffraction (XRD,Rigaku RINT-2000)with Cu K ␣radiation.Structure of the composites was characterized by FTIR.Morphology of the as-prepared composites was observed by field emission scanning electron microscope (FESEM JSM-6700)and transmission elec-tron microscope (TEM JEM-2010).Thermogravimetry-differential scanning calorimetry (TG-DSC,NETZSCH 409PC)was involved in0378-7753/$–see front matter © 2010 Elsevier B.V. All rights reserved.doi:10.1016/j.jpowsour.2010.11.1326952X.Liang et al./Journal of Power Sources196 (2011) 6951–6955determining the sulfur contents in the S/T-PPy composites.The N2 sorption measurement was performed using Micromeritics Tris-tar3000at77K,and specific surface area was calculated using the Brunauer–Emmett–Telley(BET).The mixtures of50wt.%composite,30wt.%acetylene black and 20wt.%PEO binder were added into acetonitrile to form uniform slurries.The slurries were cast onto aluminum foils.After the sol-vent evaporated,the electrodefilm was cut to sheets with14mm in diameter and further dried at50◦C under vacuum for12h.The pris-tine sulfur cathode with30wt.%sulfur,50wt.%acetylene black and 20wt.%PEO binder was prepared by the same way for comparison. CR2025type coin cells were assembled in a glove box with oxygen and water contents less than1ppm.A solution of1M LiCF3SO3dis-solved in TEGDME was employed as the electrolyte.Celgard2400 was used as the separator and lithium foils as both the counter and reference electrodes.AC impedance of the cell was measured by a Frequency Response Analyzer(FRA)technique on a Autolab Elec-trochemical Workstation over the frequency rang from1MHz to 10mHz with the amplitude of10mV.The galvanostatic charge and discharge test was carried out using a LAND CT2001A battery test system in a voltage range of1.0–3.0V vs.Li/Li+at a current density of0.1mA cm−2.3.Results and discussionFigs.1a and2a show respectively the SEM and TEM graphs of the as prepared PPyfibers.As seen,thefibers have a tubular morphology with about300nm in outer-diameter and250nm in inner-diameter.Such a structure is favorable for the loading of the molten sulfur.SEM and TEM microstructures in Figs.1a-b and2a-b show that no significant morphological difference between the S/T-PPy composite with30wt.%sulfur and the pristine tubular PPy was observed.It supposed that the sublimed sulfur was possibly restricted within the hollow of the PPy.Filling amount of sul-fur has a significant influence on the morphology of the S/T-PPy composites.Different from the composite with30wt.%sulfur,the composite with50wt.%sulfur displayed obvious irregular agglom-eration of sulfur on the outer surface of the PPy tubes,as shown in Figs.1c and2c,indicating the saturation of the sublimed sulfur for the hollow of the PPy tubes.The locations of sulfur in the composites were also roughly proved by BET surface area measurements.Surface areas of the tubular PPy or composites were analyzed through N2adsorp-tion/desorption measurements at77K,as shown in Fig.3.As the PPy tube was incorporated with sulfur,the surface area decreased obviously.The calculation based on density of sulfur (2.07g cm−3)and pore volume of the tubular PPy(0.241cm3g−1) shows that1g of PPy tube can accommodate0.498g sulfur (2.07g cm−3×0.241cm3g−1).It ensures a maximum sulfur con-tent of33.2wt.%in the PPy tube.Although parts of the sulfur could be located outer of the PPy tube as the sulfur in the S/T-PPy com-posite is large than33.2%,large amount of sulfur should possibly befilled into the PPy tube.Fig.4shows the XRD patterns of the sublimed sulfur and the S/T-PPy composites with different amounts of sulfur.As seen,the S/T-PPy composite with30wt.%sulfur has a very broad reflection peak, indicating an amorphous feature of the sulfur in the composite. During the co-heating process at150◦C,the molten sulfur could possibly penetrate into the hollow PPy of the nano-tubes due to the capillary force and the strong absorbability of PPy to sulfur, whichfinally formed a homogenous composite[16].At a higher temperature,e.g.at300◦C,sulfur coated on the outer surface of the PPy tubes would evaporate.However,when the sulfur content was higher than30wt.%,part of the sulfur crystallized on the outer surface of the tubular PPy.Fig.3b shows the reflection peak of the crystalline sulfur of the S/T-PPy composite with50wt.%sulfur.Fig.1.SEM micrographs of the T-PPy(a),the S/T-PPy composite with30wt.%sulfur (b)and the S/T-PPy composite with50wt.%sulfur(c).FTIR measurement was further conducted on the composites and the tubular PPy to indicate a possible structure change of the PPy matrix during the co-heating process with sulfur.As shown in Fig.5,all the three samples exhibited the characteristic bands of PPy,involving the pyrrole ring fundamental vibration at1545cm−1 and1458cm−1,the C–H in-plane vibration at1291cm−1and 1043cm−1and the C–N stretching vibration at1175cm−1.This is consistent with Ref.[14].It is indicated that no chemical reaction between sulfur and PPy happened during the co-heating process.The discharge curves of the S/T-PPy composite with30wt.%sul-fur are shown in Fig.6.Two voltage plateaus at about2.4V andX.Liang et al./Journal of Power Sources196 (2011) 6951–69556953Fig.2.TEM micrographs of the T-PPy(a),the S/T-PPy composite with30wt.%sulfur (b)and the S/T-PPy composite with50wt.%sulfur(c).Fig.3.Nitrogen sorption isotherms of tubular PPy and the S/T-PPy composites; inserts shows the surface areas and porevolumes.Fig.4.XRD patterns of the sublimed sulfur(a),the S/T-PPy composite with50wt.% sulfur(b)and the S/T-PPy composite with30wt.%sulfur(c).Fig.5.FTIR spectra of the T-PPy(a),the S/T-PPy composite with30wt.%sulfur(b) and the S/T-PPy composite with50wt.%sulfur(c).6954X.Liang et al./Journal of Power Sources196 (2011) 6951–6955Fig.6.Discharge curves of the S/T-PPy composite with 30wt.%sulfur.2.0V were observed for all the cycles.The 2.4V plateau is caused by the changes from element sulfur to higher-order lithium poly-sulfides (Li 2S n ,n ≥4).The 2.0V plateau is related to the reduction of higher-order polysulfides to lower-order polysulfides (n <4)[17].As seen,the composite shows a good reversibility with an initial discharge capacity as high as ca.1151.7mAh g −1.The favorable electrochemical performances of the composite could be attributed to the as-proposed microstructure,with nano-sized sulfur and the highly dispersive lithium polysulfides trapped in the hollow of the PPy tubes.Fig.7shows the cycling performance of the S/T-PPy compos-ites and the pristine sulfur electrodes.As seen,the cycling stability of the S/T-PPy composite with 30wt.%sulfur is obviously superior to that of the composite with 50wt.%sulfur and the pristine sul-fur electrode.The composite with 30wt.%sulfur remained a stable capacity of ca.650mAh g −1for over 80cycles.On the contrary,the reversible capacities at the 80th cycle for the pristine sulfur and the composite with 50wt.%sulfur were only ca.208and 140mAh g −1,respectively.As discussed above,for the S/T-PPy composite with 50wt.%sulfur,large amount of sulfur could cover the surface of the PPy tube.It could hinder the electron path for the active sulfurandFig.7.Cycling performance of the composites and the pristine sulfurelectrodes.Fig.8.AC impedance spectra of the S/T-PPy composite with 30wt.%sulfur and pure sulfur electrodes.therefore speed the capacity fading.Moreover,lithium polysulfides generated from the outer surface sulfur would easily diffuse into the electrolyte,which finally leads to a poor electrochemical behavior.On the other hand,most of the sulfur was homogenously dispersed in the PPy tube for the S/T-PPy composite with 30wt.%sulfur.The trapped sulfur and the generated polysulfides in the tube were dif-ficult to diffuse out of the tube by capillary forces.It suggested that a suitable content and the location of sulfur would be necessary for a stable cycling performance.Fig.8shows the EIS analysis results of the S/T-PPy composite and the pristine sulfur electrode after different cycles.The semi-circle at high frequency corresponds to the contact and charge trans-fer resistances.The short inclined line in low frequency region is related to the ion diffusion within the electrodes.It is obvious that both the contact and charge transfer resistances of the S/T-PPy com-posite with 30wt.%sulfur were smaller than those of the pristine sulfur electrode,which could be attributed to the enhanced con-ductivity and the favorable distribution of the nano-sized sulfur in the T-PPy pared with the pristine sulfur electrode,the charge transfer resistance of the S/T-PPy composite is much more stable upon cycling.This ensured an improved cycling performance of the composite.4.ConclusionSulfur was penetrated into the tubular PPy fibers by capillary forces during a co-heating process.The conductivity of the compos-ite was significantly improved by the tubular PPy matrix.An initial discharge capacity of ca.1151.7mAh g −1is displayed for the S/T-PPy composite with 30wt%sulfur,and nearly a constant capacity of ca.650mAh g −1after 80cycles.It was suggested that the improve-ment of the cycling stability of the S/T-PPy composite was mainly attributed to the improved conductivity,the favorable distribution of the nano-sized sulfur in the tubular PPy matrix and the excellent retention of the polysulfides within the electrode.AcknowledgementsThis work was financially supported by NSFC Project No.50730001and 50973127;research projects of Chinese Science and Technology Ministry No.2007BAA07B01and No.2007CB209700;research projects from the Science and Technology Commission of Shanghai Municipality No.08DZ2210900and No.09PJ1410800.X.Liang et al./Journal of Power Sources196 (2011) 6951–69556955References[1]W.Zheng,Y.W.Liu,X.G.Hu,C.F.Zhang,Electrochim.Acta51(2006)1330.[2]D.Marmorstein,T.H.Yu,K.A.Striebel,F.R.McLarnon,J.Hou,E.J.Cairns,J.PowerSources89(2000)219.[3]N.Petr,M.Klaus,K.S.V.Santhanam,H.Otto,Chem.Rev.97(1997)207.[4]C.Delacourt,P.Poizot,J.M.Tarascon,C.Masquelier,Nature Mater.4(2005)254–260.[5]H.Yamin,E.Peled,J.Power Sources9(1983)281.[6]X.L.Ji,K.T.Lee,L.F.Nazar,Nature Mater.8(2009)500.[7]J.Wang,S.Y.Chew,Z.W.Zhao,S.Ashraf,D.Wexler,J.Chen,S.H.Ng,S.L.Chou,H.K.Liu,Carbon46(2008)229–235.[8]L.X.Yuan,J.K.Feng,X.P.Ai,Y.L.Cao,S.L.Chen,H.X.Yang,mun.8(2006)610–614.[9]Y.V.Mikhaylik,J.R.Akridge,J.Electrochem.Soc.151(11)(2004)A1969–A1976.[10]C.D.Liang,N.J.Dudeny,J.Y.Howe,Chem.Mater.21(2009)4724–4730.[11]B.Zhang,i,Z.Zhao,X.P.Gao,Electrochim.Acta54(2009)3708–3713.[12]L.X.Yuan,H.P.Yuan,X.P.Qiu,L.Q.Chen,W.T.Zhu,J.Power Sources189(2009)1141–1146.[13]J.Wang,J.Chen,K.Konstantinov,L.Zhao,S.H.Ng,G.X.Wang,Z.P.Guo,H.K.Liu,Electrochim.Acta51(2006)4634–4638.[14]M.M.Sun,S.C.Zhang,T.Jiang,L.Zhang,J.H.Yu,mun.10(2008)1819–1822.[15]X.M.Yang,Z.X.Zhu,T.Y.Dai,Y.Lu,Macromol.Rapid Commun.26(2005)1736–1740.[16]i,X.P.Gao,B.Zhang,T.Y.Yan,Z.Zhou,J.Phys.Chem.C113(2009)4712–4716.[17]D.Marmorstein,T.H.Yu,K.A.Striebel,E.J.Cairns,J.Power Sources89(2000)219.。