地铁车站深基坑毕业设计(含外文翻译)
地铁车站基坑毕设设计
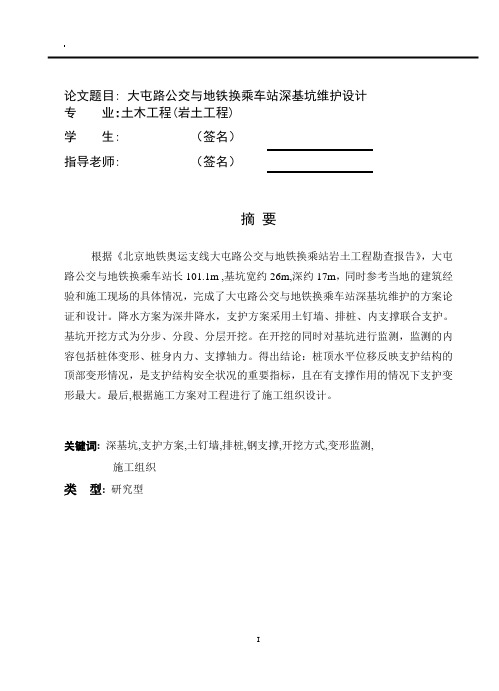
论文题目: 大屯路公交与地铁换乘车站深基坑维护设计专业:土木工程(岩土工程)学生: (签名)指导老师: (签名)摘要根据《北京地铁奥运支线大屯路公交与地铁换乘站岩土工程勘查报告》,大屯路公交与地铁换乘车站长101.1m ,基坑宽约26m,深约17m,同时参考当地的建筑经验和施工现场的具体情况,完成了大屯路公交与地铁换乘车站深基坑维护的方案论证和设计。
降水方案为深井降水,支护方案采用土钉墙、排桩、内支撑联合支护。
基坑开挖方式为分步、分段、分层开挖。
在开挖的同时对基坑进行监测,监测的内容包括桩体变形、桩身内力、支撑轴力。
得出结论:桩顶水平位移反映支护结构的顶部变形情况,是支护结构安全状况的重要指标,且在有支撑作用的情况下支护变形最大。
最后,根据施工方案对工程进行了施工组织设计。
关键词: 深基坑,支护方案,土钉墙,排桩,钢支撑,开挖方式,变形监测,施工组织类型: 研究型Subject : Deep foundation pit retaining Design of Beijing subway stations and bus transfer stationSpecialty : Civil Engineering (Geotechnical Engineering)Name : (Signature)Instructor : (Signature)ABSTRACTIn the thesis, based on 《The Geotecnical Engineering Investigation Report Of Beijing subway stations and bus transfer station》, the length of station of Beijing subway stations and bus transfer station is 101.1m, the width of foundation pit is about 26m and the depth of foundation pit is approxomately 17m. Simultaneously, refer to the local construction experience and special details of constraction site, that plan testify and design of deep foundation pit retaining of Beijing subway stations and bus transfer station has completed. The plan of retaining adopts soil-nail wall,line-pile,steel props to retain together. The type of excavation adopts a way for the minute step,the partition,the lamination excavates. While excavating foundation pit, foundation pit monitoring is carrying on, including pile deformation, pile interal force, inner axial force. The study shows the deformation characteristics of supporting structure is related to the horizontal displacement of the retaining pile top, which is the important criterion of the safety of condition of supporting structure. With the effect of supporting structure, the deformation is the largest . Finally, according to construction scheme, the construction organization design has been finished.Key Words: deep foundation pit; retaining plan; soil-nail; line-pile; steel props;excavation type; deformation; monitoring; construction organization Type : Research详细摘要本拟建工程设计说明书共分5章,包括:岩土工程勘察,基坑维护设计,基坑变形监测方案设计、施工组织设计等主要依据(1)《大屯路公交与地铁换乘车站岩土工程勘察报告》(2)《混凝土结构设计规范》(GBJ146-90)(3)《钢结构设计规范》(GB50012-2003)(4)《建筑桩基技术规范》(JGJ 94-94)(5)《建筑基坑支护技术规程》(JGJ120-1999)(6)《挤扩支盘灌注桩技术规程》(CECS192:2005)工程概况拟建大屯路公交与地铁换乘车站位于规划的奥林匹克公园中心区域,在地铁奥林匹克公园站北侧并紧邻此站,呈南北走向,是北京地铁奥运支线与大屯路隧道地下公交车站相交的节点工程,大屯路公交与地铁换乘车站共为地下两层,总长101.1m(K2+222.041~K3+323.141),南北两端断面宽24.7m、中间断面宽26.7m,均为两层三跨框架结构,本工程预期160天完成.基坑维护设计这一部分主要包括五部分。
外文翻译译文

一例开挖临近铁路深基坑的历史案例张华林、张国林、杨军生著张华林:中南大学土木建筑学院博士,同时也是学校的电子物理学讲师。
邮箱zhanghualin0703@张国林:中南大学土木建筑学院博士,中铁南方发展有限公司总工程师。
现居中国杨军生:中南大学土木建筑学院教授,邮箱jsyang@内容摘要:民智车站基坑位于深圳地铁与平南铁路相邻的地方。
其顶部有很明显的不对称荷载以及火车的动荷载影响。
因此,必须密切关组基坑开挖对铁路和基坑本身的影响,引进了深基坑紧邻铁路建设的主要技术措施,这些措施包括使用钢管桩和预应力群锚加固铁路路堤。
在建筑物里设置隔水墙,在隔水墙的中间设置预应力缆索,使用套筒式管进行注浆加固等等。
监测铁路路基的沉降以及墙的水平位移,监测结果表明,这些技术措施可以确保铁路的正常运行和坑本身的安全。
介绍:随着城市发展。
现在出现了越来越多的深基坑,而深基坑的安全问题我们应该时刻注意。
因为经济,技术,管理方面的原因,在深基坑的施工中,出现过很多事故。
分析国内深基坑事故表明,最直接的影响因素是支撑结构的稳定性这,这是导致事故的主要原因,而基坑失稳通常发生在设计和施工。
因此,如何确保深基坑的安全性,可靠性与经济理性,在城市复杂环境条件下,尽可能减少深基坑事故和损失,已经越来越需要我们去关注隧道管理、新型技术和创新民智车站基坑的特点是动态荷载和不对称荷载的综合影响,,而其地质条件和环境条件也是非常复杂的。
目前,很少有研究具有明显的不对称荷载的基坑。
目前,没有也详细的相关规范“保留和保护技术规范构建基础开挖”基坑设计和施工的铁路动态荷载以及在铁路动态荷载的影响下车站的结构功能。
因此,有巨大的价值进行研究,设计和施工的基坑紧邻铁路与不对称荷载,这是一个相当复杂的环境。
综述民智车站站位于屏南县铁路和民智路路口。
车站长度为254.6米。
左线长度为315.8米,平台有10米宽。
车站在标准宽度为19.5米,最大宽度有30米。
地铁车站毕设任务书

天津城建大学毕业设计任务书土木工程学院 10级城市地下空间工程专业 1 班姓名魏华一学号 10210118 设计题目:天津地铁6号线理工大学站主体结构设计完成期限:自2014 年 3 月日至2014 年 6 月日止指导教师:教研室/系主任:院长:学生签字:批准日期:接受任务日期:年月日注:任务书填写一式两份,一份留教研室,一份发给学生,任务完成后附入说明书内。
一.毕业设计目的及意义毕业设计是培养学生综合能力和实践能力的重要环节,根据城市地下空间工程专业的培养目标要求及毕业生的就业去向,制定本毕业设计任务书。
通过本次毕业设计,学生可将所学的专业知识综合应用于实际工程设计中,使理论与工程实践相结合,提高工程设计和实践能力;使学生在应用现行规范、标准、技术指标与经济指标等方面得到基本训练,达到对所学专业知识进行巩固、综合掌握和灵活运用的目的,提高学生独立分析和解决问题的能力。
二.毕业设计基本内容和要求:基本内容:1、车站站位选择;2、车站总平面布置(包括站位选择、出入口布置、通风亭布置等);3、车站结构形式选择;4、车站纵断面设计;5、主体结构各工况内力组合计算;6、截面检算与结构配筋设计;7、施工方案设计。
基本要求:1、设计内容要有依据;2、独立完成上述各项内容;3、论文写作规范化;4、引用规范应注明;5、每项计算应附正规的计算简图和内力图。
三.毕业设计重点研究的问题:1、车站总平面布置;2、车站主体结构横断面设计;3、车站主体结构纵断面设计;4、结构各工况内力组合计算及配筋设计;5、施工方案设计。
四.毕业设计依据、规范、规程设计依据:1、工程概况(见附件1)2、岩土工程勘察报告(见附件1)规范、规程:1、《地铁设计规范》(GB 50157-2003)2、《铁路隧道设计规范》(TB 10003-2005 J117-2005)3、《建筑结构荷载规范》(GB 50009-2012)4、《混凝土结构设计规范》(GB 50010-2010)5、《钢结构设计规范》(GB 50017-2003)6、《人民防空工程设计规范》(GB 50225-2005)7、《地下铁道工程施工及验收规范》(GB 50229-1999)8、《混凝土结构工程施工质量验收规范》(GB 50204-2002)9、《岩土工程技术规范》(DB29-20-2000)10、《铁路工程抗震设计规范》(GB 50111-2006)11、《建筑抗震设计规范》(GB 50011-2001)五.毕业设计应完成的工作:1、开题报告;2、中英文摘要;3、设计正文,包括计算说明书(规范化要求见附件2),计算分析采用手算,辅以专业软件进行计算;4、毕业设计实习报告;5、英文翻译一篇,附上英文原稿,内容限地下工程方面,字数不少于10000英文字符;6、查阅相关文献不少于20篇;7、提交图纸不少于7张,手绘或者CAD,内容包括车站总平面布置图、车站主体结构横断面图、车站主体结构纵剖面图、车站主体结构配筋图、施工方案设计图。
地铁车站深基坑围护结构设计与施工

城市化进程不断推进,带动轨道交通行业的快速发展,在地铁站建设数量增多的同时,也产生一定的建设难点,如地铁站施工区域的地基稳定性问题,部分区域的地基承载力不足,在施工过程中存在较大风险,使地铁围护结构设计施工的难度不断增大。
基于此,为保障地铁工程的顺利落实,应重视工程设计和施工管理。
当前地铁深基坑施工中的施工方案技术越发丰富,贾皓方[1]通过运用复合式防护技术提高深基坑的稳定性,景勇等[2]对不同深基坑围护结构施工方法进行对比,进一步提高地铁深基坑工程项目施工质量。
本文创新点在于结合当前地铁车站深基坑围护结构的设计要点,提出三种围护结构施工方法,并在此基础上提出设计和施工中存在的问题,全面提高工程设计和施工质量,对相关工程的设计和施工建设具有较好的借鉴作用。
1 深基坑设计原则及技术标准地铁工程的主要作用是缓解城市交通拥挤情况,因此一般建设在相对繁华的地段,而为减少对地上空间的占用,大多地铁站位于地下,这使得地铁工程在设计和施工过程中,需要向地下进行深度挖掘和设计,在该过程中为保障施工的安全性和地下车站及隧道等的稳定性,需要构建围护结构,保证工程建设效果。
因此,在深基坑设计过程中应坚持安全性原则,同时为提高工程建设效率,在施工过程中应坚持高效性原则。
此外,在深基坑工程设计和施工的各个环节应贯彻执行国家相关技术标准,做好相应的勘察与监测,从而确保基坑的安全使用与维护管理。
摘要 为解决地铁车站深基坑工程施工建设中存在的问题,文章结合实际的地铁车站工程案例进行分析,结合深基坑设计原则及技术标准和地铁车站深基坑围护结构设计要点,探究了不同深基坑围护结构设计方案的优势和不足,尝试根据案例要求选择相应的施工方案,根据方案分析介绍地铁深基坑围护结构中地下连续墙的施工工艺流程、设备选型标准、成槽工艺技术、泥浆搅拌方法及接头处施工等技术,进一步分析深基坑围护结构设计与施工中存在的问题,总结提升地铁车站深基坑围护结构设计施工质量管理的具体措施,有效提升工程建设质量。
深基坑支护毕业设计
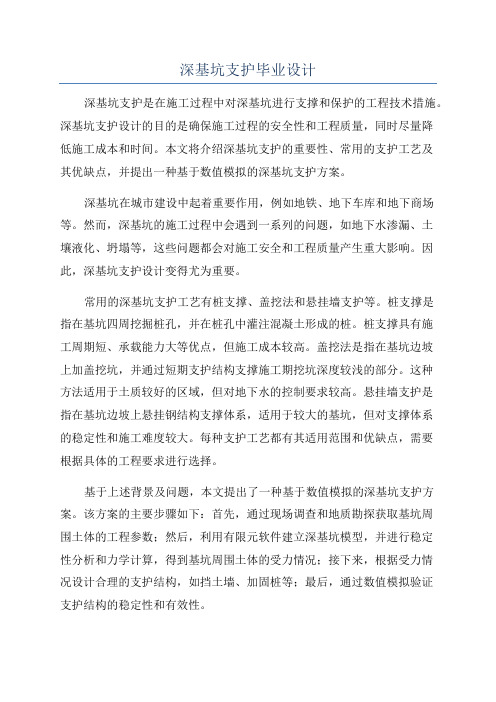
深基坑支护毕业设计深基坑支护是在施工过程中对深基坑进行支撑和保护的工程技术措施。
深基坑支护设计的目的是确保施工过程的安全性和工程质量,同时尽量降低施工成本和时间。
本文将介绍深基坑支护的重要性、常用的支护工艺及其优缺点,并提出一种基于数值模拟的深基坑支护方案。
深基坑在城市建设中起着重要作用,例如地铁、地下车库和地下商场等。
然而,深基坑的施工过程中会遇到一系列的问题,如地下水渗漏、土壤液化、坍塌等,这些问题都会对施工安全和工程质量产生重大影响。
因此,深基坑支护设计变得尤为重要。
常用的深基坑支护工艺有桩支撑、盖挖法和悬挂墙支护等。
桩支撑是指在基坑四周挖掘桩孔,并在桩孔中灌注混凝土形成的桩。
桩支撑具有施工周期短、承载能力大等优点,但施工成本较高。
盖挖法是指在基坑边坡上加盖挖坑,并通过短期支护结构支撑施工期挖坑深度较浅的部分。
这种方法适用于土质较好的区域,但对地下水的控制要求较高。
悬挂墙支护是指在基坑边坡上悬挂钢结构支撑体系,适用于较大的基坑,但对支撑体系的稳定性和施工难度较大。
每种支护工艺都有其适用范围和优缺点,需要根据具体的工程要求进行选择。
基于上述背景及问题,本文提出了一种基于数值模拟的深基坑支护方案。
该方案的主要步骤如下:首先,通过现场调查和地质勘探获取基坑周围土体的工程参数;然后,利用有限元软件建立深基坑模型,并进行稳定性分析和力学计算,得到基坑周围土体的受力情况;接下来,根据受力情况设计合理的支护结构,如挡土墙、加固桩等;最后,通过数值模拟验证支护结构的稳定性和有效性。
数值模拟可以有效地分析基坑支护过程中的各种问题,如土体的变形、应力分布和支护结构的受力情况。
通过这种方法,可以根据实际情况优化支护结构,提高工程的安全性和可靠性。
同时,数值模拟还可以节约大量的资源和时间,提高工程的经济效益。
综上所述,深基坑支护设计是保障施工安全和工程质量的重要环节。
本文介绍了常用的深基坑支护工艺,并提出了一种基于数值模拟的深基坑支护方案,以期为深基坑支护毕业设计提供一些参考和启示。
地铁车站基坑工程施工方案毕业设计
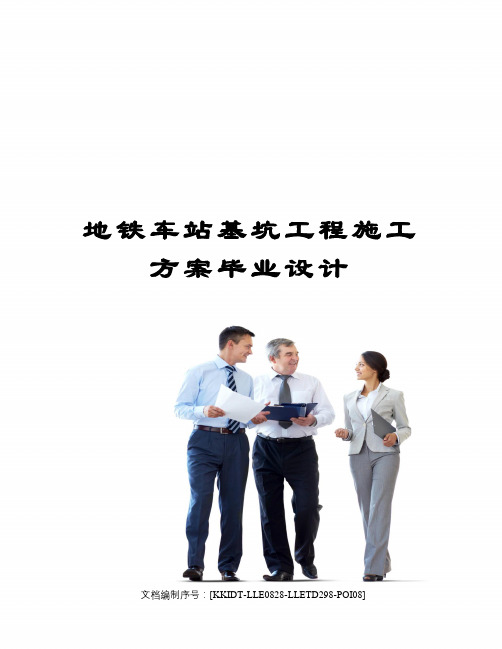
地铁车站基坑工程施工方案毕业设计文档编制序号:[KKIDT-LLE0828-LLETD298-POI08]目录苏州轨道6号线标土建工程地铁车站A深基坑工程专项方案一、编制依据本工程施工的设计图纸和设计技术要求;本工程地质勘察报告;本工程基坑周边管线图;本工程施工组织设计;施工规范及标准《地下铁道工程施工及验收规范》(GB50299——1999(2003版))《混凝土结构工程施工质量验收规范》(GB50204-2002(2011版))《建筑地基基础工程施工质量验收规范》(GB50202-2002)《建筑地基处理技术规范》(JGJ79-2012)《建筑基坑支护技术规程》(JGJ120-2012)《建筑桩基技术规范》(JGJ94-2008)《建筑结构荷载规范》(GB50009-2012)《建筑与市政降水工程技术规范》(JGJ/T111-1998)《钢筋焊接及验收规程》(JGJ18-2012)《工程测量规范》(GB50026-2007)《施工现场临时用电安全技术规范》(JGJ46-2005)《混凝土结构设计规范》(GB50010-2010)《混凝土外加剂应用技术规范》(GB50119-2003)二、工程概况工程概述工程设计概况苏州市轨道交通4号线土建施工项目Ⅳ-TS-06标包含有二站二区间:十全街站、地铁车站A、乐桥站~十全街站区间、十全街站~地铁车站A区间。
地铁车站A为4号线主线的第13座车站,为地下二层岛式车站,该站位于人民路与竹辉路新市路交叉路口下,沿人民路南北向布置。
本站与规划6号线(地下三层岛式车站)呈T型岛-岛换乘,换乘节点及规划6号线端头井与车站同时实施。
车站南侧设一联络线,联络线半径为150m,明挖结构,本站预留接口。
结构外包全长,标准段外包宽度为。
车站主体结构标准段基坑开挖深度,南北端头井基坑开挖深度、,围护结构采用800mm地下连续墙;车站换乘段基坑开挖深度,围护结构采用1000mm地下连续墙。
地铁车站深基坑围护结构设计与施工
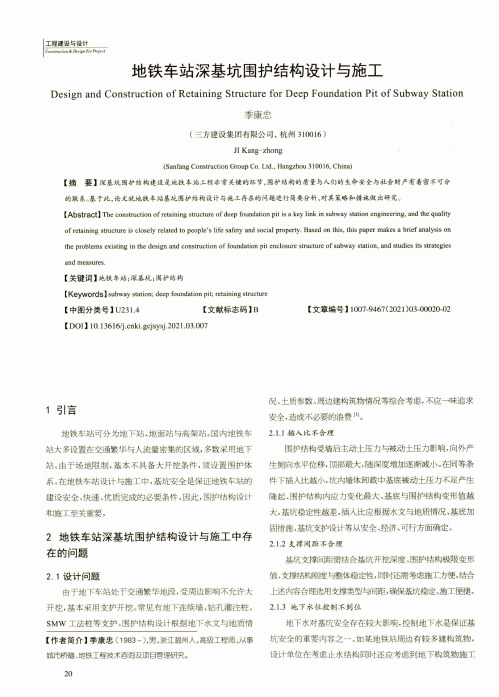
地铁车站深基坑围护结构设计与施工Design and Construction of Retaining Structure for Deep Foundation Pit of Subway Station季康忠(三方建设集团有限公司,杭州310016 )JI Kang-zhong(Sanfang Construction Group Co. Ltd., Hangzhou 310016, China)【摘要】深基坑围护结构建设是地铁车站工程非常关键的环节,围护结构的质量与人们的生命安全与社会财产有着密不可分的联系。
基于此,论文就地铁车站基坑围护结构设计与施工存在的问题进行简要分析,对其策略和措施做出研究。
【Abstract 】 The construction o f retaining structure o f deep foundation pit is a key link in subway station engineering, and the qualityo f retaining structure is closely related to people's life safety and social property. Based on this, this paper makes a brief analysis on the problems existing in the design and construction o f foundation pit enclosure structure o f subway station, and studies its strategies and measures.【关键词】地铁车站;深基坑;围护结构【K e y w o r d s 】 subway station; deep foundation pit; retaining structure【中图分类号】U 231.4 【文献标志码】B 【文章编号】1007-9467(2021 )03-00020-02【DOI 】 10.13616/j .cnki .gcjsysj .2021.03.007工程建设与设计Construction & Design For P roject1引言±也铁车站可分为地下站、地面站与高架站。
地铁隧道施工中英文外文翻译
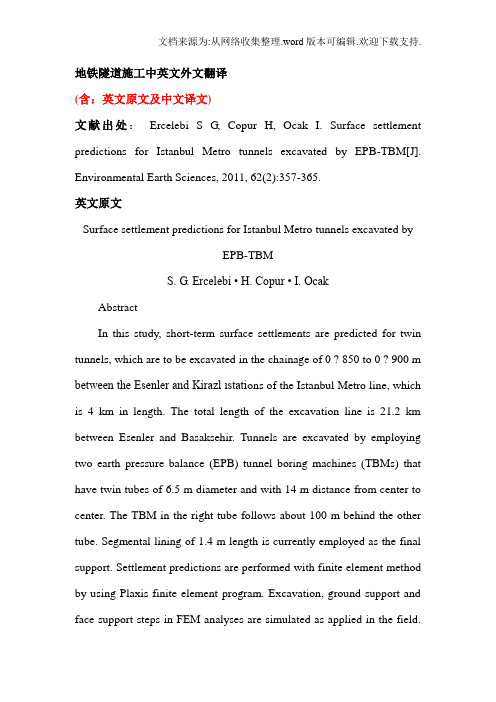
地铁隧道施工中英文外文翻译(含:英文原文及中文译文)文献出处:Ercelebi S G, Copur H, Ocak I. Surface settlement predictions for Istanbul Metro tunnels excavated by EPB-TBM[J]. Environmental Earth Sciences, 2011, 62(2):357-365.英文原文Surface settlement predictions for Istanbul Metro tunnels excavated byEPB-TBMS. G. Ercelebi • H. Copur • I. OcakAbstractIn this study, short-term surface settlements are predicted for twin tunnels, which are to be excavated in the chainage of 0 ? 850 to 0 ? 900 m between the Esenler and Kirazl ıstati ons of the Istanbul Metro line, which is 4 km in length. The total length of the excavation line is 21.2 km between Esenler and Basaksehir. Tunnels are excavated by employing two earth pressure balance (EPB) tunnel boring machines (TBMs) that have twin tubes of 6.5 m diameter and with 14 m distance from center to center. The TBM in the right tube follows about 100 m behind the other tube. Segmental lining of 1.4 m length is currently employed as the final support. Settlement predictions are performed with finite element method by using Plaxis finite element program. Excavation, ground support and face support steps in FEM analyses are simulated as applied in the field.Predictions are performed for a typical geological zone, which is considered as critical in terms of surface settlement. Geology in the study area is composed of fill, very stiff clay, dense sand, very dense sand and hard clay, respectively, starting from the surface. In addition to finite element modeling, the surface settlements are also predicted by using semi-theoretical (semi-empirical) and analytical methods. The results indicate that the FE model predicts well the short-term surface settlements for a given volume loss value. The results of semi-theoretical and analytical methods are found to be in good agreement with the FE model. The results of predictions are compared and verified by field measurements. It is suggested that grouting of the excavation void should be performed as fast as possible after excavation of a section as a precaution against surface settlements during excavation. Face pressure of the TBMs should be closely monitored and adjusted for different zones.Keywords : Surface settlement prediction, Finite element method, Analytical method , Semi-theoretical method, EPB-TBM tunneling, Istanbul MetroIntroductionIncreasing demand on infrastructures increases attention to shallow soft ground tunneling methods in urbanized areas. Many surface and sub-surface structures make underground construction works very delicate due to the influence of ground deformation, which should bedefinitely limited/controlled to acceptable levels. Independent of the excavation method, the short- and long-term surface and sub-surface ground deformations should be predicted and remedial precautions against any damage to existing structures planned prior to construction. Tunneling cost substantially increases due to damages to structures resulting from surface settlements, which are above tolerable limits (Bilgin et al. 2009).Basic parameters affecting the ground deformations are ground conditions, technical/environmental parameters and tunneling or construction methods (O’Reilly and New 1982; Arioglu 1992; Karakus and Fowell 2003; Tan and Ranjit 2003; Minguez et al. 2005; Ellis 2005; Suwansawat and Einstein 2006). A thorough study of the ground by site investigations should be performed to find out the physical and mechanical properties of the ground and existence of underground water, as well as deformation characteristics, especially the stiffness. Technical parameters include tunnel depth and geometry, tunnel diameter–line –grade, single or double track lines and neighboring structures. The construction method, which should lead to a safe and economic project, is selected based on site characteristics and technical project constraints and should be planned so that the ground movements are limited to an acceptable level. Excavation method, face support pressure, advance (excavation) rate, stiffness of support system, excavation sequence andground treatment/improvement have dramatic effects on the ground deformations occurring due to tunneling operations.The primary reason for ground movements above the tunnel, also known as surface settlements, is convergence of the ground into the tunnel after excavation, which changes the in situ stress state of the ground and results in stress relief. Convergence of the ground is also known as ground loss or volume loss. The volume of the settlement on the surface is usually assumed to be equal to the ground (volume) loss inside the tunnel (O’Reilly and New 1982). Ground loss can be classified as radial loss around the tunnel periphery and axial (face) loss at the excavation face (Attewell et al. 1986; Schmidt 1974). The exact ratio of radial and axial volume losses is not fully demonstrated or generalized in any study. However, it is possible to diminish or minimize the face loss in full-face mechanized excavations by applying a face pressure as a slurry of bentonite– water mixture or foam-processed muck. The ground loss is usually more in granular soils than in cohesive soils for similar construction conditions. The width of the settlement trough on both sides of the tunnel axis is wider in the case of cohesive soils, which means lower maximum settlement for the same amount of ground loss.Time dependency of ground behavior and existence of underground water distinguish short- and long-term settlements (Attewell et al. 1986). Short-term settlements occur during or after a few days (mostly a fewweeks) of excavation, assuming that undrained soil conditions are dominant. Long-term settlements are mostly due to creep, stress redistribution and consolidation of soil after drainageof the underground water and elimination of pore water pressure inside the soil, and it may take a few months to a few years to reach a stabilized level. In dry soil conditions, the long-term settlements may be considered as very limited.There are mainly three settlement prediction approaches for mechanized tunnel excavations: (1) numerical analysis such as finite element method, (2) analytical method and (3) semi-theoretical (semi-empirical) method. Among them, the numerical approaches are the most reliable ones. However, the results of all methods should be used carefully by an experienced field engineer in designing the stage of an excavation project.In this study, all three prediction methods are employed for a critical zone to predict the short-term maximum surface settlements above the twin tunnels of the chainage between 0 ? 850 and 0 ? 900 m between Esenler and Kirazlı stations of Istanbul Metro line, which is 4 km in length. Plaxis finite element modeling program is used for numerical modeling; the method suggested by Loganathan and Poulos (1998) is used for the analytical solution. A few different semi-theoretical models are also used for predictions. The results are compared and validated byfield measurements.Description of the project, site and construction methodThe first construction phase of Istanbul Metro line was started in 1992 and opened to public in 2000. This line is being extended gradually, as well as new lines are being constructed in other locations. One of these metro lines is the twin line between Esenler and Basaksehir, which is 21.2 km. The excavation of this section has been started in May 2006. Currently, around 1,400 m of excavation has already been completed. The region is highly populated including several story buildings, industrial zones and heavy traffic. Alignment and stations of the metro line between Esenler and Basaksehir is presented in Fig.Totally four earth pressure balance (EPB) tunnel boring machines (TBM) are used for excavation of the tunnels. The metro lines in the study area are excavated by a Herrenknecht EPB-TBM in the right tube and a Lovat EPB-TBM in the left tube. Right tube excavation follows around 100 m behind the left tube. Some of the technical features of the machines are summarized in Table.Excavated material is removed by auger (screw conveyor) through the machine to a belt conveyor and than loaded to rail cars for transporting to the portal. Since the excavated ground bears water and includes stability problems, the excavation chamber is pressurized by 300 kPa and conditioned by applying water, foam, bentonite and polymersthrough the injection ports. Chamber pressure is continuously monitored by pressure sensors inside the chamber and auger. Installation of a segment ring with 1.4-m length (inner diameter of 5.7 m and outer diameter of 6.3 m) and 30-cm thickness is realized by a wing-type vacuum erector. The ring is configured as five segments plus a key segment. After installation of the ring, the excavation restarts and the void between the segment outer perimeter and excavated tunnel perimeter is grouted by300 kPa of pressure through the grout cannels in the trailing shield. This method of construction has been proven to minimize the surface settlements.The study area includes the twin tunnels of the chainage between 0 + 850 and 0 + 900 m, between Esenler and Kirazlı stations. Gung oren Formation of the Miosen age is found in the study area. Laboratory and in situ tests are applied to define the geotechnical features of the formations that the tunnels pass through. The name, thickness and some of the geotechnical properties of the layers are summarized in Table 2 (Ayson 2005). Fill layer of 2.5-m thick consists of sand, clay, gravel and some pieces of masonry. The very stiff clay layer of 4 m is grayish green in color, consisting of gravel and sand. The dense sand layer of 5 m is brown at the upper levels and greenish yellow at the lower levels, consisting of clay, silt and mica. Dense sand of 3 m is greenish yellow and consists of mica. The base layer of the tunnel is hard clay, which is dark green,consisting of shell. The underground water table starts at 4.5 m below the surface. The tunnel axis is 14.5 m below the surface, close to the contact between very dense sand and hard clay. This depth isquite uniform in the chainage between 0 + 850 and 0 + 900 m.Surface settlement prediction with finite element modelingPlaxis finite element code for soil and rock analysis is used to predict the surface settlement. First, the right tube is constructed, and then the left tube 100 m behind the right tube is excavated. This is based on the assumption that ground deformations caused by the excavation of the right tube are stabilized before the excavation of the left tube. The finite element mesh is shown in Fig. 2 using 15 stress point triangular elements. The FEM model consists of 1,838 elements and 15,121 nodes. In FE modeling, the Mohr – Coulomb failure criterion is applied.Staged construction is used in the FE model. Excavation of the soil and the construction of the tunnel lining are carried out in different phases. In the first phase, the soil in front of TBM is excavated, and a support pressure of 300 kPa is applied at the tunnel face to prevent failure at the face. In the first phase, TBM is modeled as shell elements. In the second phase, the tunnel lining is constructed using prefabricated concrete ring segments, which are bolted together within the tunnel boring machine. During the erection of the lining, TBM remains stationary. Once a lining ring has been bolted, excavation is resumed until sufficient soilexcavation is carried out for the next lining. The tunnel lining is modeled using volume elements. In the second phase, the lining is activated and TBM shell elements are deactivated.Verification of predictions by field measurements and discussionThe results of measurements performed on the surface monitoring points, by Istanbul Metropolitan Municipality, are presented in Table 4 for the left and right tubes. As seen, the average maximum surface settlements are around 9.6 mm for the right tube and 14.4 mm for the left tube, which excavates 100 m behind the right tube. Themaximum surface settlements measured around 15.2 mm for the right tube and 26.3 mm for the left tube. Higher settlements are expected in the left tube since the previous TBM excavation activities on the right tube overlaps the previous deformation. The effect of the left tube excavation on deformations of the right tube is presented in Fig. 9. As seen, after Lovat TBM in the right tube excavates nearby the surface monitoring point 25, maximum surface settlement reaches at around 9 mm; however, while Herrenknecht TBM in the left tube passes the same point, maximum surface settlement reaches at around 29 mm.ConclusionsIn this study, three surface settlement prediction methods for mechanized twin tunnel excavations betwee n Esenler and Kirazlı stations of Istanbul Metro Line are applied. Tunnels of 6.5-m diameters with 14-mdistance between their centers are excavated by EPM tunnel boring machines. The geologic structure of the area can be classified as soft ground. Settlement predictions are performed by using FE modeling, and semi-theoretical (semi-empirical) and analytical methods. The measured results after tunneling are compared to predicted results. These indicate that the FE model predicts well the short time surface settlements for a given volume loss value. The results of some semi-theoretical and analytical methods are found to be in good agreement with the FE model, whereas some methods overestimate the measured settlements. The FE model predicted the maximum surface settlement as 15.89 mm (1% volume loss) for the right tube, while the measured maximum settlement was 15.20 mm. For the left tube (opened after the right), FE prediction was 24.34 mm, while measured maximum settlement was 26.30 mm.中文译文由EPB-TBM发掘的伊斯坦布尔地铁隧道的地表沉降预测作者:SG Ercelebi ,H Copur ,I Ocak摘要在这项研究中,预测双隧道的短期地表沉降,这些隧道将在0的里程出土。
深基坑开挖支护设计毕业论文设计

毕业设计〔论文〕题目某某地铁枣园站基坑开挖支护设计专业城市地下空间工程班级城地081学生X鹏飞指导教师X留明教授2012 年摘要基坑工程是指在地表以下开挖的一个地下空间与其配套的支护体系。
而基坑支护就是为保证基坑开挖,根底施工的顺利进展与基坑周边环境的安全,对基坑侧壁以与周边环境采用的支挡,加固与保护措施。
基坑支护体系是临时结构,安全储藏较小,具有较大风险,基坑工程具有很强的区域性。
不同水文,工程地质环境条件下基坑工程的差异很大。
基坑工程环境效应复杂,基坑开挖不仅要保证基坑本身的安全稳定,而且要有效的控制基坑周边地层移动以与保护周围环境。
本文先介绍了枣园站的工程概况,包括水文地质和周围环境,然后通过结合对现有基坑开挖支护工法和车站实际情况的比拟选择出了适合本站的开挖支护方案。
下来通过土压力的计算、结构内力的计算,配筋、验算、支撑设计、变形估算等对基坑的开挖支护作了理论上的数据分析,最后通过施工组织说明了各个工序施工的工法和应注意的问题。
关键词:支护方案,地下连续墙,支撑,施工组织设计AbstractFoundation Pit is the excavation of an underground space below the surface and a coordinated support system. Bracing of foundation pit is to ensure that excavation and foundation construction for the smooth and safe environment Foundation Pit and used the pit retaining wall reinforcement and protection.Bracing of Foundation Pit structure is the structural safety of temporary reserves are smaller, more risk. Foundation pit structure has a strong regional. Excavation works under different hydrological environmental and geological conditions are vastly. Effects plex excavation, excavation pit is not only necessary to ensure their own safety,but also to effectively control the pit surrounding strata.First,the paper introduces the general engineering situation of Zaoyuan Station,Including hydrological geology and the environment,Then,based on the existing foundation pit excavation method and station actual situation select the suitable for the station of the excavation and support scheme。
毕业设计(论文)-深基坑支护结构设计

毕业设计(论文)-深基坑支护结构设计深基坑支护结构设计是在城市建设中常见的工程项目之一。
深基坑是为了进行地下工程而开挖的大型坑穴,例如地铁站、地下商场和地下停车场等。
由于地下土壤的压力和周围环境的限制,深基坑需要进行支护结构设计来确保施工的安全性和稳定性。
本论文的目标是设计一个有效的深基坑支护结构,以应对地下土壤的压力和变形,并确保施工期间及以后的稳定性。
主要研究内容包括以下几个方面:1. 地下土壤力学特性研究:分析地下土壤的物理性质和力学特性,包括土壤的分层结构、抗剪强度、压缩性和弹性模量等。
通过土壤试验和现场勘测,获取土壤参数,并进行合理的土体模型建立。
2. 基坑支护结构类型选择:在分析和比较不同的支护结构类型后,选择最适合的支护结构类型,例如钢支撑结构、混凝土护壁结构、地下连续墙或土钉支护等。
3. 支护结构设计:根据土壤力学参数以及基坑的深度和周围环境的要求,进行支护结构的设计。
包括支撑结构的定位、类型和尺寸的确定,以及支撑结构的布置和施工方法的规划。
4. 数值模拟和分析:利用计算机软件(如PLAXIS)进行支护结构的数值模拟和分析,评估结构的稳定性和变形情况。
通过不同设计方案的比较和优化,确定最佳的支护结构设计。
5. 施工监测与控制:在施工期间,进行支护结构施工的监测和控制,确保施工过程的安全性和质量。
包括对支撑结构的变形和应力的监测,以及必要时的调整和加固。
通过以上的研究内容,可以得出一个完整的深基坑支护结构设计方案,并通过数值模拟和实际施工监测验证设计的可行性和有效性。
最终的目标是为城市建设提供一个可靠和经济的深基坑支护结构设计方案,确保施工的安全性和顺利进行。
地下室设计深基坑中英文对照外文翻译文献

地下室设计深基坑中英文对照外文翻译文献中英文对照外文翻译(文档含英文原文和中文翻译) Deep E x ca v a t ion s ABSTR ACT :All major topics in the design of in-situ retaining systems for deep excavations in urban areas are outlined. Type of wall, water related problems and water pressures, lateral earth pressures, type of support, solution to earth retaining walls, types of failure, internal and external stability problems. KEYW OR DS: deep excavation; retaining wall; earth pressure; INTR ODUCTION Numbers of deep excavation pits in city centers are increasing every year. Buildings, streets surrounding excavation locations and design of very deepbasements make excavations formidable projects. This chapter has been organized in such a way that subjects related to deep excavation projects are summarized in several sections in the order of design routine. These are types of in-situ walls, water pressures and water related problems. Earth pressures in cohesionless and cohesive soils are presented in two different categories. Ground anchors, struts and nails as supporting elements are explained. Anchors are given more emphasis compared to others due to widespread use observed in the recent years. Stability of retaining systems are discussed as internal and external stability. Solution of walls for shears, moments, displacements and support reactions under earth and water pressures are obtained making use of different methods of analysis. A pile wall supported by anchors is solved by threemethods and the results are compared. Type of wall failures, observed wall movements and instrumentation of deep excavation projects are summarized.1. TYPES OF EARTH R ETAINING WAL L S Introduction More than several types of in-situ walls are used to support excavations. The criteria for the selection of type of wall are size of excavation, ground conditions, groundwater level, vertical and horizontal displacements of adjacent ground and limitations of various structures, availability of construction, cost, speed of work and others. One of the main decisions is the water-tightness of wall. The following types of in-situ walls will be summarized below; 1. Braced walls, soldier pile and lagging walls2. Sheet-piling or sheet pile walls3. Pile walls (contiguous, secant)4. Diaphragm walls or slurry trench walls5. Reinforced concrete (cast-in-situ or prefabricated) retaining walls6. Soil nail walls7. Cofferdams8. Jet-grout and deep mixed walls9. Top-down construction 10. Partial excavation or island method Br aced Walls Excavation proceeds step by step after placement of soldier piles or so called king posts around the excavation at about 2 to 3 m intervals. These may be steel H, I or WF sections. Rail sections and timber are also used. At each level horizontal waling beams and supporting elements (struts, anchors, nails) are constructed. Soldier piles are driven or commonly placed in bored holes in urban areas, and timber lagging is placed between soldier piles during the excavation. Various details of placement of lagging are available, however, precast units, in-situ concrete or shotcrete may also be used as alternative to timber.Depending on ground conditions no lagging may be provided in relatively shallow pits. Historically braced walls are strut supported. They had been used extensively before the ground anchor technology was developed in 1970 s. Soils with some cohesion and without water table are usually suitable for this type of construction or dewatering is accompanied if required and allowed. Strut support is commonly preferred in narrow excavations for pipe laying or similar works but also used in deep and large excavations (See Fig ). Ground anchor support is increasingly used and preferred due to access for construction works and machinery. Waling beams may be used or anchors may be placed directly on soldier piles without any beams. Sheet-piling or Sheet Pile Walls Sheet pile is a thin steel section (7-30 mm thick) 400-500 mm wide. It ismanufactured in different lengths and shapes like U, Z and straight line sections (Fig. ). There are interlocking watertight grooves at the sides, and they are driven into soil by hammering or vibrating. Their use is often restricted in urbanized areas due to environmental problems like noise and vibrations. New generation hammers generate minimum vibration and disturbance, and static pushing of sections have been recently possible. In soft ground several sections may be driven using a template. The end product is a watertight steel wall in soil. One side (inner) of wall is excavated step by step and support is given by struts or anchor. Waling beams (walers) are frequently used. They are usually constructed in water bearing soils. Steel sheet piles are the most common but sometimes reinforced concrete precast sheet pile sections are preferred in softsoils if driving difficulties are not expected. Steel piles may also encounter driving difficulties in very dense, stiff soils or in soils with boulders. Jetting may be accompanied during the process to ease penetration. Steel sheet pile sections used in such difficult driving conditions are selected according to the driving resistance rather than the design moments in the project. Another frequently faced problem is the flaws in interlocking during driving which result in leakages under water table. Sheet pile walls are commonly used for temporary purposes but permanent cases are also abundant. In temporary works sections are extracted after their service is over, and they are reused after maintenance. This process may not be suitable in dense urban environment. Pile Walls In-situ pile retaining walls are very popular due to their availabilityand practicability. There are different types of pile walls (Fig. ). In contiguous (intermittent) bored pile construction, spacing between the piles is greaterthan the diameter of piles. Spacing is decided based on type of soil and level of design moments but it should not be too large, otherwise pieces of lumps etc. drop and extra precautions are needed. Cohesive soils or soils having some cohesion are suitable. No water table should be present. Acceptable amount of water is collected at the base and pumped out. Common diameters are , , m. Waling beams (usually called ?breasting beams ) are Tangent piles with grouting in between are used when secant piling or diaphragm walling equipment is not available ( in cases where ground water exists). Poor workmanship creates significant problems. Secant bored pile walls are formed bykeeping spacing of piles less than diameter (S There is also need for place for the plant. It may be constructed “hard-hard”as well as “soft-hard”. “S oft”concrete pile contains low cement content and some bentonite. Primary unreinforced piles are constructed first and then reinforced secondary piles are formed by cutting the primary piles. Pile construction methods may vary in different countries for all type of pile walls like full casing support, bentonite support, continuous flight auger (CFA) etc. mostly reinforced concrete but sheet pile sections or steel beams are also used. Diaphr agm Walls Diaphragm wall provides structural support and water tightness. It is a classical technique for many deep excavation projects, large civil engineering works, underground car parks, metro pits etc. especially under water table. Thesereinforced concrete diaphragm (continuous) walls are also called slurry trench walls due to the reference given to the construction technique where excavation of wall is made possible by filling and keeping the wall cavity full with bentonite-water mixture during excavation to prevent collapse of the excavated vertical surfaces. Wall thickness varies between m and m. The wall is constructed panel by panel in full lengths are 2 m to 10 m. Short lengths ( m) are selected in unstable soils or under very high surcharges. Nowadays depth of panels water stops exceeded 100 m, excavation depths exceeded 50 m. Different panel shapes other than the conventional straight section like T, L, H, Y, + are possible to form and used for special purposes. Panel excavation is made by cable or kelly supported buckets and by a recent designcalled ?cutter or ?hydrofraise which is a pair of hydraulically operated rotating disks provided with hard cutting tools. Excavation in rock is possible. Slurry wall technique is a specialized technique and apart from the bucket or the frame carrying the cutter equipment like crawler crane, pumps, tanks, desanding equipment, air lifts, screens, cyclones, silos, mixers, extractor are needed. Tremie concrete is placed in the slurry starting from the bottom after lowering reinforcement cages. Joint between the panels is a significant detail in water bearing soils and steel pipe, H-beam or water stops are used. R einforced C oncrete R eta ining Walls Excavation in Stages It is a common type of staged excavation wall usually supported by ground anchors. Soils with some cohesion are suitable because eachstage is first excavated before formwork and concrete placement. No water table or appreciable amount of water should be present. Sometimes micropile support is given if required due to expected cave-ins. Soil Nail Walls Similar to the method above excavation is made step by step ( to 2 m high). Shotcrete is common for facing and wiremesh is used. Soft facing is also possible making use of geotextiles. Hole is drilled, ordinary steel bars are lowered, and grout is placed without any pressure. Soil should be somewhat cohesive and no water table or significant water flow should be present. Coffer dams Cofferdam is a temporary earth retaining structure to be able to make excavation for construction activities. It is usually preferred in the coastal and sea environment like bridge piers and abutments in rivers, lakes etc., wharves, quay walls, docks, break waters andother structures for shore protection, large waterfront structures such as pump houses, subjected to heavy vertical and horizontal loads. Sheet piling is commonly used in various forms other than conventional walls like circular cellular bodies or double walls connected inside and filled with sand. Stability is maintained by sheeting driven deeper than base, sand body between sheeting and inside tie rods. Earth embankments and concrete bodies are also used. Contiguous, tangent, secant piles or diaphragm walls are constructed in circular shapes, and no internal bracing or anchoring is used to form a cofferdam. Reinforced concrete waling beams support by arching. Shafts are also made with this method. Large excavations or project details may require additional lateral support. J et Grout and Deep M ixed Walls Retainingwalls are made by single to triple row of jet grout columns or deep mixed columns. There is a soil mixed wall(SMW) technique specially developed for wall construction where H sections are used for reinforcement. Single reinforcing bar is placed in the central hole opened for jet grout columns. Anchors, nails or struts may be used for support. Top Down Constr uction Retaining structure (generally diaphragm wall) is designed and constructed as permanent load bearing walls of basement. Piles or barettes are similarly placed to complete the structural frame. Top slab is cast at the ground surface level, and excavation is made under the slab by smaller sized excavators and continued down forming basement slabs at each level. There are special connection details. Top down method is preferred in highly populated city centerswhere horizontal and vertical displacements are very critical, and anchors and struts are very difficult to use due to complex underground facilities and lifeline structures and site operations are difficult to perform. Pa r tia l Excavation or Island M ethod It is possible to give strut support to retaining walls at a later stage after constructing central sections of a building in large size excavations. Core of the structure is built at the central part making sloped excavations at peripheral areas and then the core frame is used to give support to walls (Figure ). It may be more practical and construction time may be less compared to conventional braced system. This method may not be suitable in soft and weak soils due to stability and deformation problems during sloped excavations. 2. EARTH PR ESSUR ES ON IN-SITU R ETAININGWAL L S Introduction Earth pressures on in-situ retaining walls are rather different than those on ordinary retaining walls due to the supporting elements. Free displacement of walls are not allowed. Type of support affects the distribution of earth pressure. Strut loads were measured in strutted excavations in many countries in the past, and recommendations were given. Ground anchor technology is relatively new, and data on instrumented anchored walls for total lateral。
地铁车站深基坑英文缩写
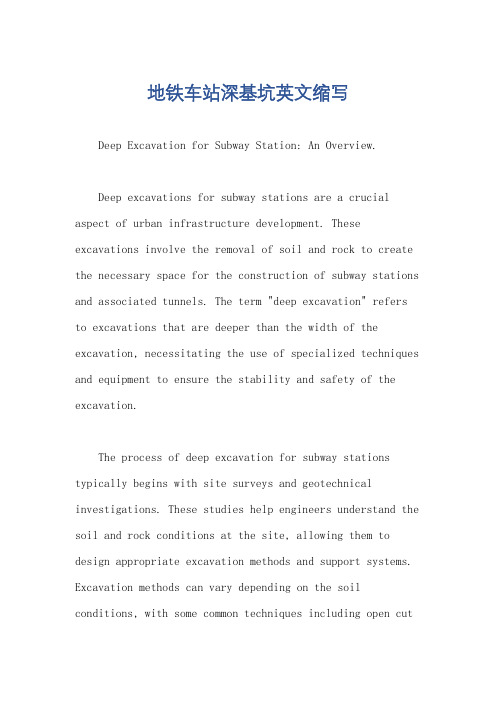
地铁车站深基坑英文缩写Deep Excavation for Subway Station: An Overview.Deep excavations for subway stations are a crucial aspect of urban infrastructure development. These excavations involve the removal of soil and rock to create the necessary space for the construction of subway stations and associated tunnels. The term "deep excavation" refers to excavations that are deeper than the width of the excavation, necessitating the use of specialized techniques and equipment to ensure the stability and safety of the excavation.The process of deep excavation for subway stations typically begins with site surveys and geotechnical investigations. These studies help engineers understand the soil and rock conditions at the site, allowing them to design appropriate excavation methods and support systems. Excavation methods can vary depending on the soil conditions, with some common techniques including open cutexcavation, shoring, and bracing.Open cut excavation is the most straightforward method, involving the removal of soil and rock using excavators or other heavy equipment. This method is typically used in areas with stable soil conditions where the risk of collapse is low. However, in areas with poor soil conditions or high groundwater levels, shoring and bracing may be necessary to support the excavation walls and prevent collapse.Shoring involves the installation of steel beams or other support structures along the excavation walls. These beams transfer the lateral pressure exerted by the soil or rock to a more stable part of the excavation, preventing collapse. Bracing, on the other hand, involves the use of steel frames or braces to provide additional support to the excavation walls. Bracing is often used in conjunction with shoring to further enhance the stability of the excavation.During the excavation process, it is crucial to monitor and control the displacement and deformation of theexcavation walls. This is achieved through the use of various monitoring instruments such as inclinometers, extensometers, and settlement gauges. By closely monitoring these parameters, engineers can detect any potential instability issues and take corrective measures promptly.In addition to ensuring the stability of the excavation, it is also important to consider the impact of excavationon the surrounding environment. Excavation activities can cause ground settlements and displacements that can affect nearby buildings, roads, and utilities. To mitigate these effects, engineers often use soil nailing, pile foundations, or other foundation enhancement techniques to improve the bearing capacity of the soil and reduce the risk of settlement.Once the excavation is complete, construction of the subway station can begin. This typically involves the installation of station walls, roofing, and otherstructural elements. The construction process must adhereto strict safety standards to ensure the safety of workers and the public.In conclusion, deep excavations for subway stations are a complex and challenging aspect of urban infrastructure development. They require careful planning, advanced engineering techniques, and strict safety measures to ensure the stability and safety of the excavation as well as the surrounding environment. With the increasing demand for subway systems in cities worldwide, the importance of deep excavations in subway station construction cannot be overstated.。
地下室设计深基坑中英文对照外文翻译文献

中英文对照外文翻译(文档含英文原文和中文翻译)Deep E x ca v a t i on sABSTR ACT :All major topics in the design of in-situ retaining systems for deep excavations in urban areas are outlined. Type of wall, water related problems and water pressures, lateral earth pressures, type of support, solution to earth retaining walls, types of failure, internal and external stability problems.KEYW OR DS: deep excavation; retaining wall; earth p ressure;INTR O DUCTIONN umbe rs of deep e x cavat i on pits i n c ity cent e r s a re incre a sing eve r y year. Buildings, streets surrounding excavation locations and design of very deep basements make excavations formidable projects. This chapter has been or ga n iz ed in suc h a w ay t hat s ubj e cts rel a ted t o deep excava t i o n project s a r e summarized in several sections in the order of design routine. These are types of in-situ walls, water pressures and water related problems. Earth pressures i n c ohesionless and coh e sive soil s are pr e s e nted in two differe nt categories. Ground anchors, struts and nails as supporting elements are explained. Anchors are given more emphasis compared to others due to widespread use obs erve d in the r e cent years. Stability of reta i ning s ystems a re discus s e d a s internal and external stability. Solution of walls for shears, moments, displacements and support reactions under earth and water pressures are obt ained m aking use o f different m e thods of a nalysis. A pile wal l supported by anchors is solved by three methods and the results are compared. Type of wall failures, observed wall movements and instrumentation of deep e xcav a tio n p roj e ct s ar e sum m arized.1. TYPES OF EARTH R ETAINING WAL L S1.1 IntroductionMore than several types of in-situ walls are used to support excavations. The criteria for the selection of type of wall are size of excavation, ground conditions, g roundwate r level, ve rtic a l a nd horizonta l displa c em e nts of adjacent ground and limitations of various structures, availability of construction, cost, speed of work and others. One of the main decisions is the wa t er-t ightness o f wal l. T he foll ow i n g types of in-sit u wa l ls will be summarized below;1.Braced walls, soldier pile and lagging walls2.Sh ee t-piling or s he et pile w a ll s3.Pile walls (contiguous, secant)4.Diaphragm walls or slurry trench walls5.Reinforced concre t e (c as t-i n-s i t u o r pr e f abricate d) r e t ai ni n g walls6.Soil nail walls7.Cofferdams8.J et-gr out and deep mixed w a ll s9.Top-down construction10.Partial excavation or island method1.1.1Br aced Wall sExcavation proceeds step by step after placement of soldier piles or so called king posts around the excavation at about 2 to 3 m intervals. These may be steel H, I or WF sections. Rail sections and timber are also used. At each level horizontal waling beams and supporting elements (struts, anchors,nails) are constructed. Soldier piles are driven or commonly placed in bored holes in urban areas, and timber lagging is placed between soldier piles dur ing the e xc a vatio n. Various det a il s o f pl a c e ment of l a gg ing are a v ailable, however, precast units, in-situ concrete or shotcrete may also be used as alternative to timber. Depending on ground conditions no lagging may be provi ded in relatively shallow pi t s.Historically braced walls are strut supported. They had been used extensively before the ground anchor technology was developed in 1970 s. S oi l s w i t h s om e c ohe s i on and w i thout wat e r table a re us u al l y suitable for t hi s type of construction or dewatering is accompanied if required and allowed. Strut support is commonly preferred in narrow excavations for pipe laying or s i m il a r works but also used i n de ep an d l ar g e ex c avat i on s (See Fig 1.1). Ground anchor support is increasingly used and preferred due to access for construction works and machinery. Waling beams may be used or anchors m a y b e place d di rec t ly on soldier pi l es w i thout a ny beam s.1.1.2Sheet-piling or Sheet Pile WallsSheet pile is a thin steel section (7-30 mm thick) 400-500 mm wide. It is m anufacture d i n different lengt hs a n d sh a pes like U, Z and stra i ght li ne sections (Fig. 1.2). There are interlocking watertight grooves at the sides, and they are driven into soil by hammering or vibrating. Their use is often restricted in urbanized areas due to environmental problems like noise and vibrations. New generation hammers generate minimum vibration anddisturbance, and static pushing of sections have been recently possible. In soft ground several sections may be driven using a template. The end product i s a w aterti g ht s t eel wall i n s oi l. O ne s i de (inner) of wal l is ex ca vate d s t ep b y step and support is given by struts or anchor. Waling beams (walers) are frequently used. They are usually constructed in water bearing soils.S teel s he et pi le s are the mo s t common but som e ti m es rein f orc ed concrete precast sheet pile sections are preferred in soft soils if driving difficulties are not expected. Steel piles may also encounter driving di ff i culties in ve ry de ns e, s t i ff soil s or in soils with boulde rs. Je t ting m ay be accompanied during the process to ease penetration. Steel sheet pile sections used in such difficult driving conditions are selected according to the driving r e s is t ance rather tha n the desi g n mo m e nt s i n the proj e ct. Ano t her fre q uent ly faced problem is the flaws in interlocking during driving which result in leakages under water table. Sheet pile walls are commonly used for t empora r y purposes but pe rm anent cas es are also a bund an t. In tem por ary works sections are extracted after their service is over, and they are reused after maintenance. This process may not be suitable in dense urban e nvironment.1.1.3Pile WallsIn-situ pile retaining walls are very popular due to their availability and practicability. There are different types of pile walls (Fig. 1.3). In contiguous (intermittent) bored pile construction, spacing between the piles is greaterthan the diameter of piles. Spacing is decided based on type of soil and level of design moments but it should not be too large, otherwise pieces of lumps e tc. d rop a n d extra preca u tions are nee de d. Coh es i ve soils or soils ha ving some cohesion are suitable. No water table should be present. Acceptable amount of water is collected at the base and pumped out. Common diameters a re 0.60, 0.80, 1.00 m. W a l ing be am s (us ually call e d …bre a s t in g be a ms ) a r e Tangent piles with grouting in between are used when secant piling or diaphragm walling equipment is not available (i.e. in cases where ground w ater exis t s). P oor w ork ma ns hi p create s significa nt proble m s.Secant bored pile walls are formed by keeping spacing of piles less than diameter (S<D). It is a watertight wall and may be more economical c ompared to di a phragm w all in small to me di um scale ex cava t ion s due t o cost of site operations and bentonite plant.There is also need for place for the plant. It may be constructed“hard-h ard”a s well as “soft-hard”.“S oft”concrete pile cont a ins low c ement content and some bentonite. Primary unreinforced piles are constructed first and then reinforced secondary piles are formed by cutting the primary piles. P i l e c ons t ruc ti on m et hods m a y var y in di f fere nt count ri es for a ll type of p ile walls like full casing support, bentonite support, continuous flight auger(CFA) etc. mostly reinforced concrete but sheet pile sections or steel beams are also used.1.1.4Diaphr agm WallsDiaphragm wall provides structural support and water tightness. It is a classical technique for many deep excavation projects, large civil engineering works, unde r ground car parks, m et ro pits et c. e s pec i ally unde r water table. These reinforced concrete diaphragm (continuous) walls are also called slurry trench walls due to the reference given to the construction technique where exc av a t ion of wal l i s m ad e possible by fi l ling and keeping the wall ca vi ty full with bentonite-water mixture during excavation to prevent collapse of the excavated vertical surfaces. Wall thickness varies between 0.50 m and 1.50 m. The w a ll is c o nstruc t ed pa nel b y p an el i n ful l dept h.Pa ne l le ng t hs are 2 m to 10 m. Short lengths (2-2.5 m) are selected in unstable soils or under very high surcharges. Nowadays depth of panels water stops exceeded 100 m, ex c avati on d ep t hs e xc e ede d 50 m. D i f fere nt pa ne l shapes o t he r t han the conventional straight section like T, L, H, Y, + are possible to form and used for special purposes. Panel excavation is made by cable or kelly supported bu c kets and by a r e cent design c alled …c u t t er o r …hydrof r a i se w hi ch is a pair of hydraulically operated rotating disks provided with hard cutting tools. Excavation in rock is possible. Slurry wall technique is a specialized tec hn i que a nd apart fro m the bucket or t he f rame carrying t h e cutter equipment like crawler crane, pumps, tanks, desanding equipment, air lifts, screens, cyclones, silos, mixers, extractor are needed. Tremie concrete is placed in the slurry starting from the bottom after lowering reinforcementcages. Joint between the panels is a significant detail in water bearing soils and steel pipe, H-beam or water stops are used.1.1.5R einforc e d C onc r ete R eta ining Walls Ex cava ti on i n StagesIt is a common type of staged excavation wall usually supported by ground anchors. Soils with some cohesion are suitable because each stage is f irst excavated be fo re fo r mwork a nd concrete p la cem e nt. No wa t e r table or appreciable amount of water should be present. Sometimes micropile support is given if required due to expected cave-ins.1.1.6S oi l Nai l Wal l sSimilar to the method above excavation is made step by step (1.5 to 2 m high). Shotcrete is common for facing and wiremesh is used. Soft facing is a lso poss i ble making us e of geo t ex t il e s. Ho l e i s dri ll e d, ordina r y steel bars are lowered, and grout is placed without any pressure. Soil should be somewhat cohesive and no water table or significant water flow should be pr e s ent.1.1.7Coffer damsCofferdam is a temporary earth retaining structure to be able to make e xcava t ion for const r uc t ion a c ti vi ties. It is usually preferr ed i n t he coast a l a nd sea environment like bridge piers and abutments in rivers, lakes etc., wharves, quay walls, docks, break waters and other structures for shore protection, large waterfront structures such as pump houses, subjected to heavy vertical and horizontal loads. Sheet piling is commonly used in various forms otherthan conventional walls like circular cellular bodies or double walls connected inside and filled with sand. Stability is maintained by sheeting dr iven de eper than ba s e, sand body between s heet i ng and inside t i e r o ds. Earth embankments and concrete bodies are also used. Contiguous, tangent, secant piles or diaphragm walls are constructed in circular shapes, and no i nte r na l brac i ng or an c horing i s us e d t o form a c o ff e rdam. R einforced concrete waling beams support by arching. Shafts are also made with this method. Large excavations or project details may require additional lateral s upport.1.1.8J et Grout and Deep M ixed WallsRetaining walls are made by single to triple row of jet grout columns or de ep mi x ed col um ns. There is a soil mi x ed wall(S M W) technique s p ecially developed for wall construction where H sections are used for reinforcement. Single reinforcing bar is placed in the central hole opened for jet groutc olum ns. Anchors, nails or struts ma y b e used fo r support.1.1.9Top Down Constr uctionRetaining structure (generally diaphragm wall) is designed and c onstr uc te d a s permane n t l o ad beari n g walls of ba s ement. Piles or barette s are similarly placed to complete the structural frame. Top slab is cast at the ground surface level, and excavation is made under the slab by smaller sized excavators and continued down forming basement slabs at each level. There are special connection details. Top down method is preferred in highlypopulated city centers where horizontal and vertical displacements are very critical, and anchors and struts are very difficult to use due to complex und erg round fa c ilitie s a nd l ifeline str uc ture s and s i t e ope ra tions are di fficult to perform.1.1.10Pa r tia l Excavation or Island M ethodI t i s poss i ble t o give s t ru t support to re tain i ng wa ll s a t a later sta ge aft e r constructing central sections of a building in large size excavations. Core of the structure is built at the central part making sloped excavations at pe ripher a l areas a nd then the c ore f rame is used t o g i ve support t o w alls (Figure 1.9). It may be more practical and construction time may be less compared to conventional braced system. This method may not be suitable in s oft and weak s oi ls du e t o stabi l ity and deformation probl e ms duri n g s l op ed excavations.2.EA R TH P R ESSU R ES ON IN-SIT U R ETAI N ING W A L L S2.1.IntroductionEarth pressures on in-situ retaining walls are rather different than those on ord inary re ta ining wall s due t o the s upporting ele m ents. Fr e e di splace m ent of walls are not allowed. Type of support affects the distribution of earth pressure. Strut loads were measured in strutted excavations in many countries in the past, and recommendations were given. Ground anchor technology is relatively new, and data on instrumented anchored walls for total lateralpressure and for water pressure are being accumulated. Earth pressure diagrams on strutted and anchored walls are expected to be somewhat di ff e re nt due t o s t i ffer s upport condi t ions in t he forme r. T heore t ical approaches will also be discussed.2.2.Ea r th Pressure Distr ibutions on WallsTerz aghi and Peck (1967) and Peck (1969) based on lo a d measurements on struts recommend the pressure distribution shown in Figure 2.1 for cohesionless soils. It is a uniform pressure and given by Eq. 2.1;p = 0.65 K A γt H 2.1where K A is the active earth pressure coefficient, H is the height of wall.Unit weight (γt) is described as the bulk unit weight in the original references.S i nce br a c ed e xcava t ions we r e gene r ally d ewate r e d in the past p r ojec t s the unit weight in the expression was described as wet or bulk. If wall is watertight and water table is present, buoyant unit weight should be usedun de r wa t er table a nd wate r pressure should be a d ded.The rectangular diagram proposed in the figure is not an actual pressure distri b uti on but an e nvel op e obt ai ned by pl ot t i ng t h e me a sured strut l oa ds converted to pressure distribution at each stage of excavation including the final depth covering all distributions. It is also called apparent pressure distribution. It is regarded as a conservative approach because strut loads calculated by such an envelope are generally greater than the measured loads.Rectangular envelope with p = 0.2 γt H is also recommended by Twine and Roscoe (1996) based on more recent field measurements. Similarly use of s ubm er g ed uni t w e i ght below wat e r t abl e a nd add i ti on of wat e r press ure i s recommended. Data on cohesive soils are classified for soft to medium stiff clays and stiff clay.A nc hor or na il supported walls may s how hig h e r l a te r al di s pl a cements, and stress increases at the upper levels of walls may be somewhat less compared to the distributions on strutted walls. However, there are no doc umented c om parisons. In t he soluti o n of a nchored wa l ls b y f init e element, boundary element, finite difference softwares or simpler spring models the analyses may be repeated without assigning pre-tensions initially like in case of nai l s upported walls a nd then a s si g n t he ca l culat e d r e actions a s pre-tensions.There are also recommendations on selection of the type of distribution i n re lation t o h e ight o f br aced walls. Dis t ributions ba s ed on pre s sure cell records are recommended for all heights but distributions by strut load measurements are not found suitable for walls higher than 15 m, they may be us e d for w a ll s of 10 –15 m he i g ht dependin g on c ondi t ions of the g r ound and construction and recommended for heights less than 10 m.Another common case is an alluvial profile where clay, silt, sand layers mixed in different proportions lie in different thicknesses. If a dominant layer is present one of the above distributions may be selected, otherwise atheoretical approach like Coulomb’s earth pressure expression may be followed making use of effective parameters, submerged unit weights and a dd e d wat e r pr essure.Effect of different surcharge loads on walls may be calculated by stress distributions in elastic medium (e.g. NAVFAC 1982). For the upper limit of ve r y rig i d wal l s the distributions are doub l ed. W ide s ur charge loa ds m ay a lso be converted to equivalent heights of soil layer.3.SUPPORTING EL EM ENTS3.1Ground A n chor s3.1.1IntroductionGround anchor is a common type of supporting element used in the de sign and const r uction of in-situ r e t ain i ng w al ls. It i s an inst al la t ion that i s capable of transmitting an applied tensile load to a load bearing stratum which may be a soil or rock. A summary about ground anchors will be given i n this s e ct i on. Typ e s, ca pa city, de s ig n, construction a nd qual it y c ontrol w ill be reviewed.3.1.2Types and C apa city of Anchor sTem porary anc h or a nd perma ne n t a nc ho r are the main types and as the names imply the former is used in temporary works and usually a period of maximum two years are assigned as the design life. Design life of a permanent anchor is the same as the life of structure. Corrosion protection details and factors of safety are the main differences between the two types.Free length is a function of height of the wall. Fixed length is selected according to type of soil and it varies between 3 m and 10 m. Fixed length is t he t ensile load bea r ing part of a n anc hor in s oi l. The r e a r e diff e rent mechanisms of stress transfer from the fixed anchor zone to surrounding ground. It is usually referenced as …bond stress and depends on soil type a nd gr out in g pro c edure. Except i ng sp e cia l c on struct i ons i n f i xe d pa rt of anchors like under reams in stiff clays, jet grouted bodies or inflated aluminum bags, most common type of construction is cement (and water) g rout wi t h som e ad dit i ves. V ery stiff, hard so i ls and ro m an c hettecks m ay be grouted without pressure. Many soils may be grouted but grouting pressure,water cement ratio (w /c) and additives play major role depending on the permea bi lity and s tif f nes s of the soil. Fi x ed le n gt h of ancho r enlarges i n diameter with increasing grout pressure. Grout permeates or fractures or pushes the soil around depending on type of soil, grout and pressure level. Coars e and fine g r a ined gra nul a r s oi ls, alluvi a l soil s a nd we a k r oc ks are generally grouted with several bars of pressure through casing or using packer. Stiff cohesive soils and fine cohesionless soils may be grouted at hig he r press ur es (greater t han 15-20 b ars) to for m hi ghl y f r actur e d larger fixed end bodies to obtain higher capacities. Post-grouting techniques through tube and manchette (sleeve tubing) or double/triple tubing are used. Main possibilities in failure of a single anchor are failure of ground/grout interface, tendon itself or grout/tendon interface.Capacity of anchors in cohesionless soils depends on average grain size (D50), uniformity coefficient (CU), relative density (RD), diameter of drill hole, method of grout injectio n (pr i m ary/sec on dary) and g rout pre s sure.Higher D50, CU, RD and grout pressure result in higher capacities. Fixed lengths of 4 to 8 m are in use and 6 m seems to be a lower limit of r e comme nda ti on for fine to m e dium sands, and the lower limit may be l e s s for gravelly soils. Permeability and grout characteristics (i.e. water-cement ratio, pressure) are key factors for capacities. At lower pressure levels (less than 1 M P a) a nd higher p ress u re s (more t h an 2 MPa) capacit i es fr om 400/500to 1400/1700 kN are observed in fine to medium sands and dense coarser sands and gravels respectively. This wide range is due to enlargement of the dr i ll hole an d m o re grout i n trusion in coarser soil s. Cal cu lat i ons by s oi l mechanics principles cannot explain these capacities. Best way is to perform tests on design anchors.L oa d c a pac i ty of anc ho rs in cl a ys is low c om pared to sandy a nd grave l ly soils. Fixed anchor lengths in design are usually 7-8 m. Application of low grouting pressure (less than 1 MPa) and use of casing tubes may be beneficial to t he capacity. Casin g tube s a lso prevent f ormation of r e mol de d soft cohesive film on borehole surface in layered soils which reduces capacity significantly. Capacity of anchors can be increased in stiff fissured clays using high pressure grouting and post-grouting. High pressure causes hydrofracturing and/or penetration of grout into existing fissures. Using bellsor under-reams in the fixed anchor zone in stiffer clays (cU>90 kPa) also increases capacity. Tremie grouted straight shafts in very stiff or hard soils yiel d suffic i ent c ap acities s i milar to anch or s in rock. Skin fric t ion (m) increases with decreasing plasticity and increasing consistency ((w L- w)/IP). m range is from 50 to more than 400 kPa in stiff clays. Pressure grouting is a lso used in rock. Skin fric t ion or bond v al ues f or va riety of r ocks c an be found in BS(8081) and other references.Grout is in tension like the tendon, and it is assumed that ultimate bond s t re ss bet w een g r out and te ndon is uni f orm. For c lea n stra n ds and defor m ed bars a limit of 2 MPa is recommended. Bond strength can be significantly affected by the surface condition of the tendon, particularly when loose and l ubric ant ma t eri a ls or loose rus t, soil, p ai nt ar e pr es e n t a t t he i nt e rfac e. Minimum grout compressive strength of 30 MPa is recommended prior to stressing. At grout/encapsulation interface maximum ultimate bond is taken 3 M Pa. Enc a psulations are usua l ly used i n permanent an c hor appl i cations against corrosion, and single or double (concentric) corrugated plastic or metal ducts cover single or multi-unit tendons and grouted. Details at head, fr ee l e ngth, seal b et w een fre e and fi x ed len g ths and fixed le ng th vary in m a ny different patented designs (See for example FIP,1986).3.1.3Planning of Anchor sFree length at each excavation stage and fixed length are selected. Fixed length in cohesionless and cohesive soils has been discussed in the previoussection. It is usually kept constant in a project. Fixed length has to be placed outside the active wedge behind wall. It is customary to add an extra to free l ength. Thi s is e s pe c i al l y useful i n projec t s i n stiff cl a ys where deformations at the back of wall extend to distances three times the depth of excavation. Minimum spacing of anchors should be 1.5-2 m and minimum distance of 2-3 m should b e provided between the fixed l e ng ths. A n an c hor d ensity of3-8 m2/anchor generally observed in projects depends on factors such as water pressure, type of soil, depth of excavation etc. If closely spaced a nchor s a re use d ei t her a dj a cent anchor s are de s igned a t di fferent angles w i t h the horizontal like 10°and 15°or identical rows are not used. Angles between 5°and25°w ith the horizontal are normally selected unless fixed l eng t hs ar e locat e d in deeper c o m petent laye r s. Two anchors may be pla c ed at the same anchor head at different angles if required. It is considered a good practice to design positions of fixed lengths in a disorderly manner. Another r e com me nd ati o n i s to k ee p the whole f i x ed length i n a s ingle la ye r i n layered soils if possible. Distance of fixed length to any adjacent foundation/underground service is recommended 3 m minimum. Spacing of a nchors is c ont rolle d by type of wall, and vert i c a l dista nc e betwee n rows is determined by a trial and error process (i.e. anchor capacity vs. spacing, reaction forces etc.).深基坑工程摘要本文概述了城市中保留原址的深基础连续墙系统。
深基坑支护毕业设计
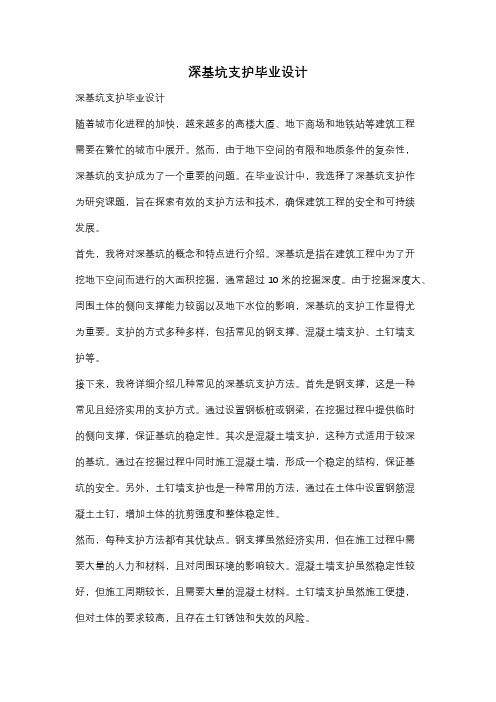
深基坑支护毕业设计深基坑支护毕业设计随着城市化进程的加快,越来越多的高楼大厦、地下商场和地铁站等建筑工程需要在繁忙的城市中展开。
然而,由于地下空间的有限和地质条件的复杂性,深基坑的支护成为了一个重要的问题。
在毕业设计中,我选择了深基坑支护作为研究课题,旨在探索有效的支护方法和技术,确保建筑工程的安全和可持续发展。
首先,我将对深基坑的概念和特点进行介绍。
深基坑是指在建筑工程中为了开挖地下空间而进行的大面积挖掘,通常超过10米的挖掘深度。
由于挖掘深度大、周围土体的侧向支撑能力较弱以及地下水位的影响,深基坑的支护工作显得尤为重要。
支护的方式多种多样,包括常见的钢支撑、混凝土墙支护、土钉墙支护等。
接下来,我将详细介绍几种常见的深基坑支护方法。
首先是钢支撑,这是一种常见且经济实用的支护方式。
通过设置钢板桩或钢梁,在挖掘过程中提供临时的侧向支撑,保证基坑的稳定性。
其次是混凝土墙支护,这种方式适用于较深的基坑。
通过在挖掘过程中同时施工混凝土墙,形成一个稳定的结构,保证基坑的安全。
另外,土钉墙支护也是一种常用的方法,通过在土体中设置钢筋混凝土土钉,增加土体的抗剪强度和整体稳定性。
然而,每种支护方法都有其优缺点。
钢支撑虽然经济实用,但在施工过程中需要大量的人力和材料,且对周围环境的影响较大。
混凝土墙支护虽然稳定性较好,但施工周期较长,且需要大量的混凝土材料。
土钉墙支护虽然施工便捷,但对土体的要求较高,且存在土钉锈蚀和失效的风险。
因此,在毕业设计中,我将综合考虑各种支护方法的优缺点,提出一种综合性的深基坑支护方案。
该方案将充分利用各种支护技术的优势,减少其缺点,以达到经济、安全和环保的目标。
例如,可以结合钢支撑和混凝土墙支护的方式,根据基坑的具体情况选择合适的方案。
同时,可以引入新的材料和技术,如纤维增强材料和数值模拟分析等,提高支护的效果和施工的效率。
最后,我将通过实地调研和数值模拟分析等方法,对所提出的深基坑支护方案进行验证和优化。
地铁深基坑支护设计毕业设计
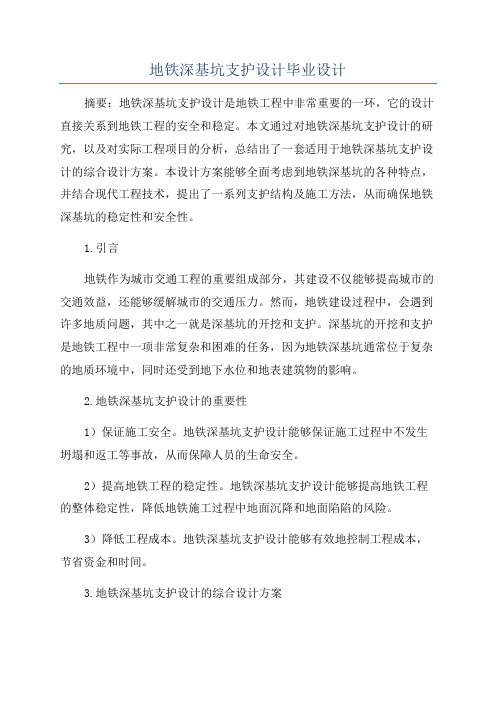
地铁深基坑支护设计毕业设计摘要:地铁深基坑支护设计是地铁工程中非常重要的一环,它的设计直接关系到地铁工程的安全和稳定。
本文通过对地铁深基坑支护设计的研究,以及对实际工程项目的分析,总结出了一套适用于地铁深基坑支护设计的综合设计方案。
本设计方案能够全面考虑到地铁深基坑的各种特点,并结合现代工程技术,提出了一系列支护结构及施工方法,从而确保地铁深基坑的稳定性和安全性。
1.引言地铁作为城市交通工程的重要组成部分,其建设不仅能够提高城市的交通效益,还能够缓解城市的交通压力。
然而,地铁建设过程中,会遇到许多地质问题,其中之一就是深基坑的开挖和支护。
深基坑的开挖和支护是地铁工程中一项非常复杂和困难的任务,因为地铁深基坑通常位于复杂的地质环境中,同时还受到地下水位和地表建筑物的影响。
2.地铁深基坑支护设计的重要性1)保证施工安全。
地铁深基坑支护设计能够保证施工过程中不发生坍塌和返工等事故,从而保障人员的生命安全。
2)提高地铁工程的稳定性。
地铁深基坑支护设计能够提高地铁工程的整体稳定性,降低地铁施工过程中地面沉降和地面陷陷的风险。
3)降低工程成本。
地铁深基坑支护设计能够有效地控制工程成本,节省资金和时间。
3.地铁深基坑支护设计的综合设计方案1)地质勘察和地下水分析。
通过对地下土质和地下水位的详细调查和分析,确定地铁深基坑的稳定性和安全性。
2)支护结构设计。
根据地质勘察和地下水分析结果,结合现代支护结构的设计理论和施工经验,设计出适应于地铁深基坑的支护结构,能够承受地铁施工过程中的压力和力量。
3)施工方法设计。
根据支护结构设计的要求,确定地铁深基坑的施工方法,包括挖土、护土、浇筑混凝土等,同时还需要考虑到施工过程中的安全和环境保护。
4.地铁深基坑支护设计的案例分析以城市地铁施工过程中遇到的一个深基坑为例,对地铁深基坑支护设计进行了详细的案例分析。
通过对该深基坑的地质特点、地下水位、地表建筑物等的调查和分析,结合现代支护结构的设计理论和施工经验,提出了一个适用于该深基坑的支护设计方案。
地铁一号线A标段基坑支护设计

XX 地铁一号线A 标段基坑支护设计摘要:该毕业设计以XX 地铁一号线工程为背景,自主设计了XX 地铁一号线标段1380.000K +~14200.000K +的支护结构,并对该段基坑支护工程进行了支护方案比选。
对支护结构进行内力计算和强度验算。
此外,还做了有关降水设计、基坑底稳定性分析和地下连续墙的施工工艺和质量控制。
并绘制了支护结构平面布置图、剖面图、有关详图及支撑结构的配筋图。
关键词:XX 地铁; 支护结构; 地下连续墙; 内支撑;基坑底稳定性Abstract:The graduation design to the subway line project that a backgr o u n d.In d e p e n d e n t d e s i gn s s u z h o u a n m u b e r o f t h e s u b w a y K+design optimization and construction technolo K+~14200.0001380.000gy for bracing structure of extra large reservoir. And foundation pit cutti ng of the city construction of the city better than the scheme. the city on the structure and proofing internal force. In addition, for the precipita tion design, and the foundation pit cutting of the stability analysis for t he construction process and quality control, and draws out the city flat structure, sections, the detailed map and support structure with the band s.Key words: Suzhou Subway; Underground continuous;Route alternative; The underground concatenation wall;Supporting structure in support;Foundation pit cutting of the stability;Precipitation design; the construction technology and quality controlof drilling driven cast-in-place pile绪论XX城市轨道交通规划将按照“统一规划、分步实施”的原则,以满足2015年XX市机械出行人数总量600万人次的需求。
地铁车站深基坑毕业设计(含外文翻译)

摘要毕业设计主要包括三个部分,第一部分是上海地铁场中路站基坑围护结构设计;第二部分是上海地铁场中路站基坑施工组织设计;第三部分是专题部分,盾构施工预加固技术研究。
在第一部分基坑围护结构设计中,根据场中路站基坑所处的工程地质、水文地质条件和周边环境情况,通过施工方案的比选,确定采用地下连续墙作为基坑的围护方案,支撑方案选为对撑,从地面至坑底依次设四道钢管支撑,并进行围护结构及支撑的内力计算、相应的强度和地连墙的配筋验算以及基坑的抗渗、抗隆起和抗倾覆等验算。
第二部分的施工组织设计,根据基坑围护方案、施工方法和隧道周边的环境情况,对施工前准备工作,施工场地布置,围护结构施工、基坑开挖与支撑安装等进行设计,并编制了工程进度计划,编写了相应的质量、安全、环境保护等措施。
第三部分专题内容是盾构施工中的预加固技术研究。
针对工程施工中的地质条件和施工工况,总结了盾构施工中的土体预加固的技术措施和相关的参考资料,提出在盾构施工中土体预加固的技术措施。
关键词:基坑;地下连续墙;施工组织;支撑体系;盾构预加固技术目录第一部分上海地铁场中路站基坑围护结构设计1 工程概况 (1)1.1工程地质及水文地质资料 (1)1.2工程周围环境 (2)2 设计依据和设计标准 (4)2.1 工程设计依据 (4)2.2 基坑工程等级及设计控制标准 (4)3 基坑围护方案设计 (5)3.1基坑围护方案 (5)3.2基坑围护结构方案比选 (6)4 基坑支撑方案设计 (8)4.1支撑结构类型 (8)4.2支撑体系的布置形式 (8)4.3支撑体系的方案比较和合理选定 (10)4.4基坑施工应变措施 (10)5 计算书 (12)5.1 荷载计算 (12)5.2 围护结构地基承载力验算 (14)5.3 基坑底部土体的抗隆起稳定性验算 (14)5.4抗渗验算 (15)5.5抗倾覆验算 (16)5.6整体圆弧滑动稳定性验算 (17)5.7围护结构及支撑内力计算 (17)5.8 支撑强度验算 (21)5.9 地下连续墙配筋验算 (23)6 基坑主要技术经济指标 (25)6.1 开挖土方量 (25)6.2 混凝土浇筑量 (25)6.3 钢筋用量 (25)6.4 人工费用 (25)第二部分上海地铁场中路站基坑施工组织设计1 基坑施工准备 (25)1.1 基坑施工的技术准备 (25)1.2 基坑施工的现场准备 (25)1.3 基坑施工的其他准备 (27)2 施工方案 (29)2.1 概况 (29)2.2 施工方法的确定 (29)2.3 施工流程 (32)2.4 质量控制 (35)2.5 施工主要技术措施 (36)2.6关键部位技术措施 (38)3施工总平面布置 (40)3.1 施工现场广场临时建筑物的布置原则及位置 (40)3.2 施工用的临时运输线路的布置 (40)3.4 建筑材料的堆放位置 (40)4施工进度计划及管理措施 (41)4.1 工程安排原则 (41)4.2 施工进度计划 (41)4.3 施工质量过程控制 (42)5质量、安全、文明管理措施 (43)5.1 质量管理措施 (43)5.2 土方运输环境管理规定 (44)5.3 安全生产管理措施 (44)5.4 文明施工措施 (44)第三部分盾构施工中的预加固技术研究1概述 (47)1.1盾构法概述 (47)1.2盾构法的施工条件 (47)1.3 盾构施工工艺 (47)1.4盾构法施工的优缺点 (49)1.5盾构法施工预加固的必要性 (49)2 盾构施工预加固技术 (50)2.1概述 (50)2.2冻结法 (50)2.3 注浆法 (51)2.4高压旋喷桩 (52)3 水平冻结法在盾构进洞中的应用 (54)3.1 工程概况 (54)3.2周边环境状况 (54)3. 3地基加固方式的选择 (54)3. 4水平冻结法地基加固施工 (54)3.5冻结加固的效果 (56)3.6盾构进洞存在的风险 (57)3.7盾构进洞的保证措施 (57)4.小结 (59)参考文献 (60)第四部分外文翻译翻译原文 (62)中文译文 (66)致谢 (88)第一部分上海地铁场中路站基坑围护结构设计1 工程概况上海地铁七号线一期工程二标段场中路站位于沪太公路南侧和大场税务所东侧。
- 1、下载文档前请自行甄别文档内容的完整性,平台不提供额外的编辑、内容补充、找答案等附加服务。
- 2、"仅部分预览"的文档,不可在线预览部分如存在完整性等问题,可反馈申请退款(可完整预览的文档不适用该条件!)。
- 3、如文档侵犯您的权益,请联系客服反馈,我们会尽快为您处理(人工客服工作时间:9:00-18:30)。
摘要毕业设计主要包括三个部分,第一部分是上海地铁场中路站基坑围护结构设计;第二部分是上海地铁场中路站基坑施工组织设计;第三部分是专题部分,盾构施工预加固技术研究。
在第一部分基坑围护结构设计中,根据场中路站基坑所处的工程地质、水文地质条件和周边环境情况,通过施工方案的比选,确定采用地下连续墙作为基坑的围护方案,支撑方案选为对撑,从地面至坑底依次设四道钢管支撑,并进行围护结构及支撑的内力计算、相应的强度和地连墙的配筋验算以及基坑的抗渗、抗隆起和抗倾覆等验算。
第二部分的施工组织设计,根据基坑围护方案、施工方法和隧道周边的环境情况,对施工前准备工作,施工场地布置,围护结构施工、基坑开挖与支撑安装等进行设计,并编制了工程进度计划,编写了相应的质量、安全、环境保护等措施。
第三部分专题内容是盾构施工中的预加固技术研究。
针对工程施工中的地质条件和施工工况,总结了盾构施工中的土体预加固的技术措施和相关的参考资料,提出在盾构施工中土体预加固的技术措施。
关键词:基坑;地下连续墙;施工组织;支撑体系;盾构预加固技术目录第一部分上海地铁场中路站基坑围护结构设计1 工程概况 (1)1.1工程地质及水文地质资料 (1)1.2工程周围环境 (2)2 设计依据和设计标准 (4)2.1 工程设计依据 (4)2.2 基坑工程等级及设计控制标准 (4)3 基坑围护方案设计 (5)3.1基坑围护方案 (5)3.2基坑围护结构方案比选 (6)4 基坑支撑方案设计 (8)4.1支撑结构类型 (8)4.2支撑体系的布置形式 (8)4.3支撑体系的方案比较和合理选定 (10)4.4基坑施工应变措施 (10)5 计算书 (12)5.1 荷载计算 (12)5.2 围护结构地基承载力验算 (14)5.3 基坑底部土体的抗隆起稳定性验算 (14)5.4抗渗验算 (15)5.5抗倾覆验算 (16)5.6整体圆弧滑动稳定性验算 (17)5.7围护结构及支撑内力计算 (17)5.8 支撑强度验算 (21)5.9 地下连续墙配筋验算 (23)6 基坑主要技术经济指标 (25)6.1 开挖土方量 (25)6.2 混凝土浇筑量 (25)6.3 钢筋用量 (25)6.4 人工费用 (25)第二部分上海地铁场中路站基坑施工组织设计1 基坑施工准备 (25)1.1 基坑施工的技术准备 (25)1.2 基坑施工的现场准备 (25)1.3 基坑施工的其他准备 (27)2 施工方案 (29)2.1 概况 (29)2.2 施工方法的确定 (29)2.3 施工流程 (32)2.4 质量控制 (35)2.5 施工主要技术措施 (36)2.6关键部位技术措施 (38)3施工总平面布置 (40)3.1 施工现场广场临时建筑物的布置原则及位置 (40)3.2 施工用的临时运输线路的布置 (40)3.4 建筑材料的堆放位置 (40)4施工进度计划及管理措施 (41)4.1 工程安排原则 (41)4.2 施工进度计划 (41)4.3 施工质量过程控制 (42)5质量、安全、文明管理措施 (43)5.1 质量管理措施 (43)5.2 土方运输环境管理规定 (44)5.3 安全生产管理措施 (44)5.4 文明施工措施 (44)第三部分盾构施工中的预加固技术研究1概述 (47)1.1盾构法概述 (47)1.2盾构法的施工条件 (47)1.3 盾构施工工艺 (47)1.4盾构法施工的优缺点 (49)1.5盾构法施工预加固的必要性 (49)2 盾构施工预加固技术 (50)2.1概述 (50)2.2冻结法 (50)2.3 注浆法 (51)2.4高压旋喷桩 (52)3 水平冻结法在盾构进洞中的应用 (54)3.1 工程概况 (54)3.2周边环境状况 (54)3. 3地基加固方式的选择 (54)3. 4水平冻结法地基加固施工 (54)3.5冻结加固的效果 (56)3.6盾构进洞存在的风险 (57)3.7盾构进洞的保证措施 (57)4.小结 (59)参考文献 (60)第四部分外文翻译翻译原文 (62)中文译文 (66)致谢 (88)第一部分上海地铁场中路站基坑围护结构设计1 工程概况上海地铁七号线一期工程二标段场中路站位于沪太公路南侧和大场税务所东侧。
拟建之场中路站建(构)筑物主要由地铁行车道及人行通道组成,车站段地铁行车道主体长约220m,宽约20m,人行通道长约70~80m,宽约10m。
车站结构型式为地下二层岛式,底板埋深为15.00m。
1 .1工程地质及水文地质资料1.1.1工程地质条件场中路站场地地形较平坦,地面标高在4.16m~4.65m之间。
标准段位置,土层由上至下分别为:①1填土,①2滨土,②1粉质粘土,③淤泥质粉质粘土,④淤泥质粘土,⑥粉质粘土,⑦1-1粉砂,⑧1粘土,⑧2-2粉砂夹粉质粘土,勘察成果表明,地基土分布有以下特点:1)浅部无粉性土(②3层)分布,第②1层褐黄色~灰黄色粉质粘土下为第③层淤泥质粉质粘土和第④层淤泥质粘土,其中第③层夹较多薄层粉性土。
2)场地北侧受古河道切割影响,第⑥层、第⑦1-1层缺失,分布有第⑤1层粘性土。
场地南侧为正常分布区,第④层直接与第⑥层暗绿色粉质粘土相连,第⑥层硬土层层顶埋深一般在17.4~18.4m,厚度约1.4~4.0m;第⑦1-1层层顶埋深一般在20~21m左右。
3)第⑧1、⑧2-2层顶面埋深分别为31m、45m左右。
车站所在场地范围内自上向下土层分布情况见表1.1。
表1.1 土层分布情况土层编号土层名称土层描述土层厚度(m)层底标高①1填土杂~黄褐色,很湿,松散,上部主要为混凝土地坪、碎石、煤渣等,下部由粘性土等组成。
1.52.8②1粉质粘土褐黄~灰黄色,湿~很湿,可塑~软塑,中等~高等压缩性,含氧化铁斑点及铁锰质结核,随深度增加土性渐变软。
无摇震反应,土面较光滑,韧性中等~高2.2 0.6③淤泥质粉质粘土灰色,饱和,流塑,高等压缩性,含云母、有机质,在4.0~6.0m夹较多量薄层粉性土,土质不均匀。
摇震反应很慢,土面较粗糙,3.7 -3.1韧性中等,干强度中等。
④淤泥质粘土灰色,饱和,流塑,高等压缩性,含云母、有机质及少量贝壳碎屑,夹少量薄层粉砂,土质均匀。
摇震反应无,土面光滑有油脂光泽,韧性高等,干强度高10.8 -13.9⑥粉质粘土暗绿~草黄色,湿~很湿,可塑~硬塑,中等压缩性,含氧化铁斑点及铁锰质结核,夹少量灰白色高岭土,下部夹粘质粉土。
无摇震反应,土面较光滑,韧性中等~高等,干强度中等~高等。
2.7 -16.6⑦1-1粉砂草黄色,饱和,中密度~密实,中等压缩性,含云母、少量氧化铁条纹,夹砂质粉土,上部夹薄层粘性土。
6.1 -22.71.1.2水文条件拟建场地地下水主要有浅部土层中的潜水和深部粉性土层中的(微)承压水。
据区域资料,承压水位,一般低于潜水位,浅部土层中的潜水位埋深,一般离地表面0.3~1.5m,年平均地下水位离地表面0.5~0.7m,低水位埋深为1.50m;第⑦1-1层承压水位埋深为3~11m。
潜水位和承压水位随季节、气候等因素而有所变化。
江河边一定距离范围内,特别是有浅层粉性土或砂土分布区,其潜水位受潮汐影响较明显。
据有关资料,地下水的温度,埋深在4m范围内受气温变化影响,4m以下水温较稳定,一般为16~18°。
根据地质资料,潜水水位埋深为1.23~2.80m,第⑦1-1层承压水水位埋深为4.08m。
水质分析表明,地下水对混凝土无腐蚀性。
由于拟建场地地下水水位较高,根据上海地区经验,当地下水(潜水)对混凝土无腐蚀性性时,其土对混凝土亦无腐蚀性,故判定拟建场地地下水和土对混凝土无腐蚀性。
另据水质分析报告和类同工程经验判定,场地地下水对钢结构有弱腐蚀性。
1.2工程周围环境1.2.1邻近建筑场地西侧为居民住宅,住宅离基坑较远,在基坑开挖影响范围以外。
基坑东侧为沪太路,南侧为洛场路,路面下有较多的市政管线,需在施工中加强对基坑变形的控制。
1.2.2 地下管线根据现有的管线资料显示,场中路站端头井围护结构范围内无地下管线,但在工地围场边上有一排架空电线,施工中,应避免大型机械设备接触或碰撞管线。
在基坑东侧的沪太路上,分布有上水Ø200、电话36孔、上水Ø500、上水Ø1800、雨水Ø400;在洛场路口上分布有上水Ø300、上话12孔、雨水Ø400、上话(2根光缆)、煤气Ø200。
洛场路上的管线分布较远,基坑开挖影响较小,但沪太路下的管线需加强监测和保护。
具体管线分布情况参见表1.2。
表1.2场中路管线分布详细列表道路管线种类埋深(m)至端头井基坑距离(m)沪太路电话36孔 1.0 7 上水Ø200 0.7 9 上水Ø500 1.0 10 上水Ø1800 1.7 15.2 雨水Ø400 1.2 18.2洛场路上水Ø300 1.2 超过基坑影响范围上话Ø12孔 1.0 超过基坑影响范围雨水Ø400 0.6 超过基坑影响范围上水Ø300 0.7 超过基坑影响范围上话(2根光缆) 1.0 超过基坑影响范围煤气Ø200 0.7 超过基坑影响范围备注:在至基坑外侧边缘1.5H(H为基坑开挖深度)距离内为基坑影响范围1.2.2 周围道路在场中路站南端头井位置,是沪太路、场中路及洛场路的交汇处,工程位于交汇处的西北侧,场区施工对社会的交通影响较小。
1.2.3 施工条件土的类型为中软或软弱土,建议按软弱土考虑。
建筑的场地类别为Ⅲ类,相应特征周期值为0.45S。
本场地属对建筑抗震不利地段。
周围环境开阔,交通便利,有足够的空间堆放土方、材料和混凝土等。
1.2.4 邻近地区对地面沉降很敏感的建筑资料和要求临近建筑主要为商业用房,且楼层高度都不高。
对地面沉降不是很敏感,故该条不考虑。
2 设计依据和设计标准2.1 工程设计依据本工程设计执行的规范和标准:(1)《岩土工程勘察规范》(GB 50021-2001);(2)《建筑结构荷载规范》(GB 50009-2001)(3)《建筑基坑支护技术规程》(JGJ 120-2012);(4)《建筑地基基础设计规范》(GB 50007-2011);(5)《地下铁道、轻轨交通岩土工程勘察规范》(GB 50307-1999);(6)《钢结构设计规范》(GB50017-2003);(7)《混凝土结构设计规范》(GB50010-2010);2.2 基坑工程等级及设计控制标准根据《建筑基坑支护技术规程》(JGJ120-2012)规定,基坑的侧壁安全等级分为三级,基坑支护结构设计应根据表2.1选用相应的侧壁安全等级及重要性系数。