Thermal decomposition of copolymers of methyl methacrylate and alkyl methacrylates
刚果红法测定聚偏氯乙烯(pvdc)涂料样品的初始分解温度
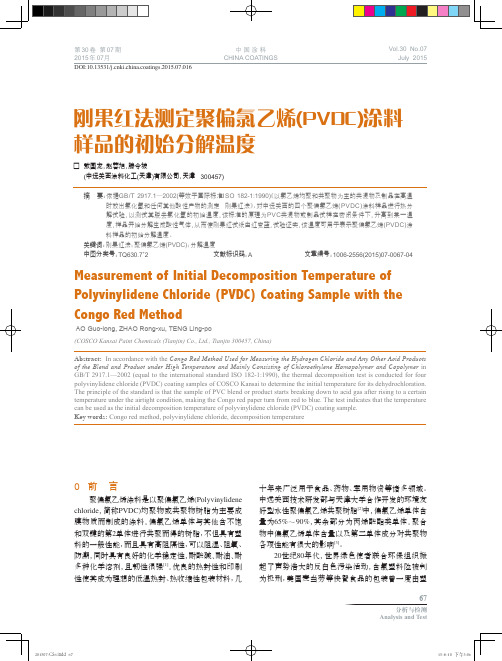
672015 年 07月DOI:10.13531/ki.china.coatings.2015.07.001第 30 卷 第 07 期 CHINA COATINGS July 2015中 国 涂 料Vol.30 No.07刚果红法测定聚偏氯乙烯(PVDC)涂料样品的初始分解温度Measurement of Initial Decomposition Temperature ofPolyvinylidene Chloride (PVDC) Coating Sample with the Congo Red MethodAO Guo-long, ZHAO Rong-xu, TENG Ling-po(COSCO Kansai Paint Chemicals (Tianjin) Co., Ltd., Tianjin 300457, China)Abstract: In accordance with the Congo Red Method Used for Measuring the Hydrogen Chloride and Any Other Acid Products of the Blend and Product under High Temperature and Mainly Consisting of Chloroethylene Homopolymer and Copolymer in GB/T 2917.1—2002 (equal to the international standard ISO 182-1:1990), the thermal decomposition test is conducted for four polyvinylidene chloride (PVDC) coating samples of COSCO Kansai to determine the initial temperature for its dehydrochloration. The principle of the standard is that the sample of PVC blend or product starts breaking down to acid gas after rising to a certain temperature under the airtight condition, making the Congo red paper turn from red to blue. The test indicates that the temperature can be used as the initial decomposition temperature of polyvinylidene chloride (PVDC) coating sample.Key words: Congo red method, polyvinylidene chloride, decomposition temperature0 前 言聚偏氯乙烯涂料是以聚偏氯乙烯(Polyvinylidene chloride ,简称PVDC)均聚物或共聚物树脂为主要成膜物质而制成的涂料。
DSC、TMA、DMA、TGA介绍

100
150
200
250
100 300
Universal V3.0G TA Instruments
Temperature (°C)
Storage Modulus (MPa)
Loss Modulus (MPa)
Heat Flow (mW )
Tan Delta
DSC: What Affects the Glass Transition?
Heat Flow (mW)
First Tg
155.93℃
Residual Cure
102.64℃ 20.38J/g
Second
Tg
100℃
150℃250℃来自250℃Temperature ℃
Thermoset:Isothermal Temp 170 Deg C Different IsOthermal test results comparison 熱固型材料在170度恆溫下不同恆溫時間對Tg之量測
Thermal decomposition (pyrolysis) of urea in an open reaction vessel
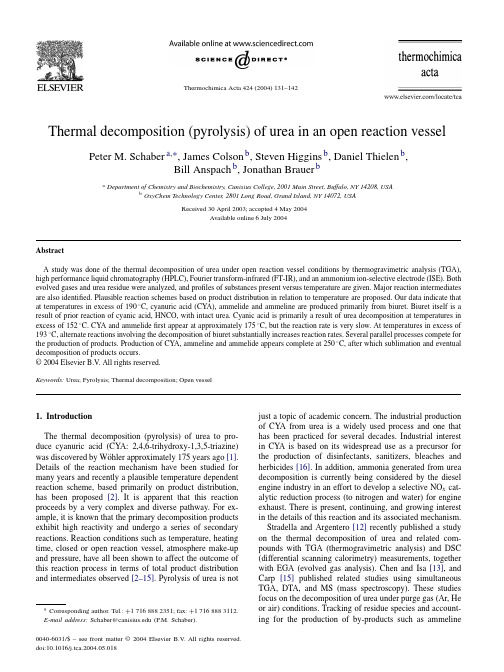
Thermochimica Acta 424(2004)131–142Thermal decomposition (pyrolysis)of urea in an open reaction vesselPeter M.Schaber a ,∗,James Colson b ,Steven Higgins b ,Daniel Thielen b ,Bill Anspach b ,Jonathan Brauer baDepartment of Chemistry and Biochemistry,Canisius College,2001Main Street,Buffalo,NY 14208,USAb OxyChem Technology Center,2801Long Road,Grand Island,NY 14072,USAReceived 30April 2003;accepted 4May 2004Available online 6July 2004AbstractA study was done of the thermal decomposition of urea under open reaction vessel conditions by thermogravimetric analysis (TGA),high performance liquid chromatography (HPLC),Fourier transform-infrared (FT-IR),and an ammonium ion-selective electrode (ISE).Both evolved gases and urea residue were analyzed,and profiles of substances present versus temperature are given.Major reaction intermediates are also identified.Plausible reaction schemes based on product distribution in relation to temperature are proposed.Our data indicate that at temperatures in excess of 190◦C,cyanuric acid (CY A),ammelide and ammeline are produced primarily from biuret.Biuret itself is a result of prior reaction of cyanic acid,HNCO,with intact urea.Cyanic acid is primarily a result of urea decomposition at temperatures in excess of 152◦C.CYA and ammelide first appear at approximately 175◦C,but the reaction rate is very slow.At temperatures in excess of 193◦C,alternate reactions involving the decomposition of biuret substantially increases reaction rates.Several parallel processes compete for the production of products.Production of CY A,ammeline and ammelide appears complete at 250◦C,after which sublimation and eventual decomposition of products occurs.©2004Elsevier B.V .All rights reserved.Keywords:Urea;Pyrolysis;Thermal decomposition;Open vessel1.IntroductionThe thermal decomposition (pyrolysis)of urea to pro-duce cyanuric acid (CY A:2,4,6-trihydroxy-1,3,5-triazine)was discovered by Wöhler approximately 175years ago [1].Details of the reaction mechanism have been studied for many years and recently a plausible temperature dependent reaction scheme,based primarily on product distribution,has been proposed [2].It is apparent that this reaction proceeds by a very complex and diverse pathway.For ex-ample,it is known that the primary decomposition products exhibit high reactivity and undergo a series of secondary reactions.Reaction conditions such as temperature,heating time,closed or open reaction vessel,atmosphere make-up and pressure,have all been shown to affect the outcome of this reaction process in terms of total product distribution and intermediates observed [2–15].Pyrolysis of urea is not∗Corresponding author.Tel.:+17168882351;fax:+17168883112.E-mail address:Schaber@ (P.M.Schaber).just a topic of academic concern.The industrial production of CYA from urea is a widely used process and one that has been practiced for several decades.Industrial interest in CY A is based on its widespread use as a precursor for the production of disinfectants,sanitizers,bleaches and herbicides [16].In addition,ammonia generated from urea decomposition is currently being considered by the diesel engine industry in an effort to develop a selective NO x cat-alytic reduction process (to nitrogen and water)for engine exhaust.There is present,continuing,and growing interest in the details of this reaction and its associated mechanism.Stradella and Argentero [12]recently published a study on the thermal decomposition of urea and related com-pounds with TGA (thermogravimetric analysis)and DSC (differential scanning calorimetry)measurements,together with EGA (evolved gas analysis).Chen and Isa [13],and Carp [15]published related studies using simultaneous TGA,DTA,and MS (mass spectroscopy).These studies focus on the decomposition of urea under purge gas (Ar,He or air)conditions.Tracking of residue species and account-ing for the production of by-products such as ammeline0040-6031/$–see front matter ©2004Elsevier B.V .All rights reserved.doi:10.1016/j.tca.2004.05.018132P .M.Schaber et al./Thermochimica Acta 424(2004)131–142and ammelide and accompanying synthetic details,were not included in these reports.In this study,an analysis of urea decomposition under open reaction vessel conditions utilizing TGA,high performance liquid chromatography (HPLC),Fourier transform-infra red (FT-IR),and an am-monium ion-selective electrode (ISE),is reported.Both evolved gases and urea residue were analyzed and profiles of substances present versus temperature are given.Major reaction intermediates are identified.Although others are considered,plausible reaction schemes based on product distribution in relation to temperature,favoring those with observable intermediates,are proposed.2.ExperimentalUrea was obtained from Aldrich Chemical Co.,Milwau-kee,WI (99%pure,ACS reagent grade),and used without further purification.Biuret was obtained from Fisher Scien-tific Co.,Pittsburgh,PA (99.9%pure,ACS reagent grade)and also used without further purification.CY A,ammelide,ammeline,and melamine were obtained from OxyChem’s industrial process and purified in-house.Urea residue in an open reaction vessel was obtained by heating 3.0g samples of urea in a 10mL Pyrex TM beaker on a sand bath until the desired temperature was reached.The sample was then quickly cooled to room temperature in a water bath and the residue collected.Chromatographic analyses of urea residues were con-ducted with HPLC methods previously described in the lit-erature [2].Samples were analyzed for the presence of urea,biuret,CY A,ammelide,ammeline and melamine.An HPLC Mass Table (Table 1)and an HPLC Mass Plot (Fig.1)were constructed from the data obtained.Thermogravimetric analysis (TGA)and measurements of mass losses (and the 1st derivative)versus temperature were determined using a Hi-Res TGA 2950Thermogravimetric Analyzer under N 2(g)purge.The “high resolution”op-tion was routinely used.Typically,30–50mg of sample was placed on a Pt pan and heated at 10◦C min −1.Urea “critical temperatures”,defined as those temperatures corresponding to plateau regions,points of rapidly changing mass,or where phase changes are known to occur (melting points,etc.),were identified from the TGA data plot (Fig.2).Table 1HPLC Mass Table (urea pyrolysis)open reaction vessel a Temperature (◦C)Mass (g)Urea (g)Biuret (g)CY A (g)Ammelide (g)Ammeline (g)Melamine (g)Total %recovery 133100.098.7 1.0––––99.719080.060.520.00.60.5––102.022533.0 6.0 4.615.6 6.10.9–100.525029.00.30.219.97.7 1.30.058101.427528.00.50.318.97.5 1.30.056102.332020.0––12.1 4.9 1.10.04490.73505.0––3.11.00.50.10094.0aThese data were calculated based on the results obtained from HPLC analysis assuming 100.0g of urea initially present.Table 2Urea pyrolysis residue analysis;ammonium ion (NH 4+)analysis with an ion-selective electrode a Temperature (◦C)Concentration of NH 4+ion (ppm)13370190380225260025026027515032512035090aResults in ppm are based upon the mass of the original sample.Table 3Urea pyrolysis off-gas;ammonium ion (NH 4+)analysis with an ion-selective electrode a Temperature range (◦C)Concentration of NH 4+ion (ppm)Room temperature to 133210133–21011900210–2258500225–2551210255–3505200350–400450aResults in ppm are based upon the mass of the original sample.Ammonium ion [NH 4+]analysis of both urea residue and pyrolysis off-gases were accomplished using an ammonium ISE (Orion,Beverly,MA).Typically for urea residues,a 1%aqueous solution was made and analyzed.Resultant con-centration versus temperature data are collected in Table 2.Urea pyrolysis off-gases were generated by placing a 3.0g sample of urea in a three-neck round bottom flask fitted with a thermometer,a thermal watch device,a N 2(g)purge,and an NH 3(g)scrubber (gas trap)consisting of 50.0mL of 12.0M HCl.As the urea sample was heated,the gases that evolved between the desired “critical temperature”points were allowed to pass through the scrubber and collected.Ammonium ion analysis was conducted on diluted scrubber solutions.Results are collected in Table 3.FT-IR spectra of the urea melt were collected using the Applied Systems Inc.(ASI)REACT-1000system (ASI SensIR Technologies,Danbury,CT),fitted with a sili-con (Si)probe.A spectrum was acquired every 2min asP .M.Schaber et al./Thermochimica Acta 424(2004)131–142133Fig.1.HPLC Mass Plot:urea pyrolysis reaction (assume 100.0g of urea initially).“critical temperature”points,between 133and 225◦C,were ramped to and held with the aid of an Omega thermocou-ple (Fig.3).Qualitative analysis was performed using the REACT-1000soft-ware package supplied by ASI.Analy-Fig.2.TGA:urea pyrolysis reaction.sis of urea pyrolysis off-gases were conducted by placing a 1.0g sample of urea into a glass vial and inserting into a tube furnace fitted with a N 2(g)purge and gas collec-tion adapter.The urea sample was heated to the desired134P .M.Schaber et al./Thermochimica Acta 424(2004)131–142Fig.3.FT-IR Si-probe spectra:urea pyrolysis reaction.“critical temperature”and held there for several minutes with the aid of a thermal watch device.Evolved gases were subsequently swept into an IR gas cell fitted with NaCl windows.FT-IR spectra of urea off-gases were obtained with a Nicolet 20SXB Spectrometer (Nicolet,Madison,WI).Condensed materials adhering to the surface of the gas collection adapter were collected and subjected to melting point determination with a 510Melting Point ap-paratus (Brinkmann Instruments,Inc.,Westbury,NY),and HPLC analysis using methods previously referenced in this section.3.Results and discussion 3.1.Process overviewThe TGA of urea measured with a heating rate of 10◦C min −1under N 2(g)purge between 50and 600◦C is given in Fig.2.Three major stages of mass loss are ob-served and calculated to be approximately 72,24,and 4%.The pyrolysis reaction of urea in an open reaction vessel can be divided into four major “reaction”regions.“Reaction”regions are dominated by different chemical processes asso-ciated with the mass loss stages observed in the TGA.What follows is a detailed description of the chemical process in each of the urea pyrolysis “reaction”regions.3.2.First “reaction”region (room temperature to 190◦C)Little significant mass loss (0.01%)is observed when heating urea in an open reaction vessel from room tem-perature to its melting point 133◦C.HPLC analysis of the residue at the melting point,gives 98.7%urea and 1.0%biuret (Table 1).The biuret present at this point primarily represents contamination in the original sample.Only a small relative amount of [NH 4+]ion is observed in both the residue and off-gas analysis (Tables 2and 3)and a very small [NCO −]absorption peak at 2156cm −1is noted in the FT-IR spectra (Si-probe)of the melt (Fig.3).These observations are consistent with a very small amount of urea decomposition and vaporization.Recently,Chen and Isa [13]have also observed only a small mass change prior to urea’s melting point,which is consistent with our data.Mass loss begins in earnest at approximately 140◦C as observed from the TGA (Fig.2).The loss observed between 140and 152◦C,is associated primarily with urea vaporiza-tion.(Condensed material adhering to the surface walls of the gas collection adapter (see Section 2),in this temper-ature range was identified as urea from melting point and HPLC determinations.)A more significant mass loss is ob-served between approximately 152and 160◦C and proceeds via two processes;continued urea vaporization and decom-position [9].P.M.Schaber et al./Thermochimica Acta424(2004)131–142135 Urea decomposition:H2N–CO–NH2(m)urea+heat→NH+4NCO−(m) ammonium cynate →NH3(g)ammonia+HNCO(g)cyanic acid(1)At152◦C decomposition begins[4],Eq.(1),accompanied by vigorous gas evolution from the melt.Above152◦C the decomposition rate of urea increases rapidly.At160◦C,the FT-IR Si-probe spectra of the melt,indicates an increase in intensity of the[NCO−]peak from that observed at140◦C (Fig.3).The FT-IR spectrum of gases evolved at160◦C in-dicates the strong presence of NH3(g)(peaks at3333,965 and930cm−1),but little discernible HNCO(g).Stradella and Argentero[12]have,however,observed HNCO(g)in the evolved gases at this temperature.Chen and Isa[13],and Carp[15]have also identified evidence of HNCO(g)pro-duction in their studies.That the ammonium cyanate salt, [NH4+NCO−],is formed as an intermediate in Eq.(1)has recently been confirmed by Carp[15].These data are all con-sistent with urea’s initial decomposition to[NH4+NCO−], which itself decomposes resulting in the evolution of NH3 (g)and HNCO(g).A product of urea decomposition,HNCO,begins to react with intact urea to produce biuret at approximately160◦C, Eq.(2).1This is supported by an increase in the intensity of a peak unique to biuret at1324cm−1,in the FT-IR Si-probe spectra(Fig.3).Biuret production:H2N–CO–NH2(m)urea +HNCO(g)cyanic acid→H2N–CO–NH–CO–NH2(m)biuret(2)Between160and190◦C urea continues to vaporize and decompose and HNCO,continues to react with intact urea to produce biuret.However,complications also begin to enter the system in this temperature range.A small amount of HNCO can now begin to react with biuret[10],Eq.(3),or itself,Eq.(4),to produce CYA,or with urea,Eq.(5),to produce ammelide.From the FT-IR Si-probe data,it appears that the production of both CY A and ammelide commence simultaneously at approximately175◦C.This is supported by an increase in the intensity of respective peaks unique to CYA at1058cm−1,and ammelide,at977cm−1,in the FT-IR Si-probe spectra(Fig.3).CYA production:H2N–CO–NH–CO–NH2(m)biuret+HNCO(g)cyanic acid →CY A(s)cyanuric acid+NH3(g)ammonia(3)via Eq.(4)is a reasonable candidate and one that has been known experimentally for many years.Herzberg and Reid1The actual reaction to form biuret likely involves the interaction of urea with[NCO−]in the melt[11].The form of the equation used here is for convenience,and will be used throughout this document.However, it should be realized that reactions in the melt involving HNCO are likely to proceed via[NCO−]interaction.[17]have observed that if HNCO(g)exceeds a critical vapor pressure,3HNCO(g)cyanic acid→CY A(s)cyanuric acid(4)spontaneous and rapid polymerization to CY A can oc-cur.This critical vapor pressure can be quite low,espe-cially in the presence of a metal surface.2Another po-tential route to CY A involves the cyclicization of triuret (H2N–CO–NH–CO–NH–CO–NH2)with the evolution of NH3[3].Chen and Isa[13]identify the existence of urea trimer under TGA conditions.However,since no triuret is observed in these studies,its existence under open reaction vessel conditions is questionable.Ammelide production:2HNCO(g)cyanic acid+H2N–CO–NH2(m)urea→ammelide(s)+2H2O(g)(5) The intermediate for Eq.(5)could be either biuret,via Eq.(2),or dicyanic acid(H2N2C2O2).Biuret is observed in the reaction sequence and if it also serves as an intermedi-ate to ammelide,Eq.(5)is then very similar in essence to that represented in Eq.(7).Dicyanic acid would be very un-stable at these temperatures and if behaving as the interme-diate,would not be expected to accumulate to a significant extent.However,since not a hint of dicyanic acid was de-tected in this study,its contribution to ammelide production is questionable under the reaction conditions imposed. The conceptually simplest route to ammelide involves the direct reaction of CY A with available NH3(ammination), Eq.(6).This is a seeming logical path but one that has been determined to occur only under conditions of high pressure [18]or temperatures above300◦C[19].In the industrial preparation of CY A using a kiln,less ammelide is in general, CY A(s)cyanuric acid+NH3(g)ammonia→ammelide(s)+H2O(g)(6)produced when NH3is removed from the system.Although this would seem consistent with Eq.(6),the situation is likely more reflective of the different environmental aspects of lab-oratory versus industrial preparation of CY A,where temper-ature gradients in the kiln may produce conditions favorable to the production of ammelide in this fashion.A more likely laboratory route is one that parallels the formation of CY A, that is,the direct reaction of biuret with HNCO,Eq.(7), but with the formation of ammelide[20,21].Other possible routes to ammelide have been suggested,however,theyH2N–CO–NH–CO–NH2(s)biuret+HNCO(g)cyanic acid→ammelide(s)+H2O(g)(7)2Under TGA conditions,or with the use of a purge gas,this route is expected to be somewhat curtailed.However,Eq.(4)may be significant in the industrial preparation of CY A where metal kilns are often used.136P.M.Schaber et al./Thermochimica Acta424(2004)131–142typically require the presence of a precursor such as ammi-nated bi-or triuret,guanidine[10],or cyanamide[19].These proposed precursor species are stable in this“reaction”re-gion and if present,evidence of their existence would be expected.Our HPLC data give no indication any exist in measurable amounts in the residue(see Table1;note ap-proximately100%recoveries at these temperatures),nor are any detected in the FT-IR data.Under the conditions imposed,the reaction scheme most likely to produce ammelide,Eq.(7),operates in parallel to the one most likely to produce CYA,Eq.(3).Both prod-ucts are produced at about the same overall rate,and begin to appear at approximately the same temperature(175◦C). This suggests the reactions producing them share common reactants,(i.e.biuret and HNCO)and possess similar acti-vation energies.In addition,since relatively small amounts of CYA and ammelide are observed in the residue(Table1), whatever route adapted,reaction rates are rather sluggish for their production in this temperature range.Up to190◦C,the net mass loss for the system is pre-dominately the result of urea decomposition.By this same temperature,urea mass has decreased by38.2%,biuret mass has increased by19.0%and has reached a maximum ac-cording to HPLC analysis(Table1).The FT-IR of evolved gases is dominated by NH3due primarily to the contin-ual and increased decomposition rate of urea.Peaks in the 2100–2300cm−1region indicate the presence of HNCO, or of volatile salts of HNCO3such as ammonium cyanate, [NH4+NCO−],or hydronium cyanate,[H3O+NCO−][22]. Continued build up of[NH4+NCO−]in the melt is indicated by both the FT-IR Si-probe data(Fig.3),and the[NH4+]ion analysis of the residue(Table2).However,although build-ing,the amount of[NH4+]ion in the residue(380ppm)is still not significantly large.These data indicate that much of the NH3formed escapes the system without further reaction and that HNCO has either reacted to produce biuret,CY A, or ammelide,or escaped the system in the vapor form.In addition,the vaporization of volatile HNCO salts,has just begun.3.3.Second“reaction”region(between190and250◦C) As temperature exceeds190◦C,alternate reaction se-quences begin to dominate the process.At approximately 193◦C,the increased evolution of gases from the urea melt reflects the beginning of biuret decomposition(biuret melts with decomposition at193◦C),Eq.(8).A corresponding mass maximum for biuret(Table1),and1st derivative peak in the urea TGA near this temperature are observed(Fig.2). However,the gas evolution rate increase is not only the result of biuret and continuing urea decomposition.3In our studies,unequivocal identification of the HNCO salts cannot be made at this temperature due to poor resolution in this region of the FT-IR spectrum.Biuret decomposition:H2N–CO–NH–CO–NH2(m)biuret→H2N–CO–NH2(m)urea+HNCO(g)cyanic acid(8)(The urea produced by biuret decomposition in Eq.(8),is unstable in this“reaction”region and will itself decompose further to HNCO(g)and NH3(g),Eq.(1)).It also results from gas producing,auto-condensation reactions associated with biuret decomposition,Eqs.(9)and(10),to produce CY A and ammelide.CY A production:2H2N–CO–NH–CO–NH2(m)biuret→CY A(s)cyanuric acid+HNCO(g)cyanic acid+2NH3(g)ammonia(9)Ammelide production:2H2N–CO–NH–CO–NH2(m)biuret→ammelide(s)+HNCO(g)cyanic acid+NH3(g)ammonia+H2O(g)(10) Although Eqs.(3),(4)and(9)all contribute to the produc-tion of CY A,Eq.(3)is expected to predominate at lower temperatures[9](first“reaction”region),Eqs.(4)and(9) become more competitive at higher temperatures(second “reaction”region)where biuret begins to decompose.It is also expected that Eq.(4)will begin to play an increased role in CY A production if,or when,critical vapor pressure is achieved[17].A similar situation exists with respect to the production of ammelide.Eq.(7)is expected to predomi-nate at lower temperatures(first“reaction”region),Eq.(10) above193◦C(second“reaction”region).However,only small amounts of CY A and ammelide are observed prior to the decomposition temperature of biuret.At this point the production rate for both CY A and ammelide increase rapidly.The close relationship between the onset of biuret decomposition and increased production rates suggests that both CY A and ammelide are produced largely from biuret and in parallel fashion within the second“reaction”region [8].In addition,Eqs.(9)and(10)involve stoichiometric larger amounts of gaseous products than reaction candidates previously considered.The large increase in gas evolution physically observed in the urea melt above193◦C and the large amount of[NH4+]detected(11,900ppm)in the py-rolysis off-gas analysis between133and210◦C(Table3) are consistent with both continued urea and biuret decom-position and the production of CY A and ammelide by ki-netically faster mechanisms.TGA and DTA data collected on biuret itself indicates ready transformation to CY A upon heating[8,23].Stradella and Argentero[12]estimate that about50%of biuret is converted to CY A.At temperatures exceeding the melting point of biuret,conversion to CY A most probably involves Eqs.(4)and(9).At lower tem-peratures an alternative reaction path,be it minor,is one reminiscent of the production of CY A from urea and in-volves the initial decomposition of biuret to urea and HNCO.P.M.Schaber et al./Thermochimica Acta424(2004)131–142137Fig.4.TGA:biuret pyrolysis reaction.(Although the melting point of biuret is193◦C,our TGA analysis indicates mass loss prior to this temperature(Fig.4). These data are consistent with other investigators including Nelson et al.[8,23]).Biuret decomposition is followed by reaction of intact biuret with HNCO to produce CY A as in the urea process.The beginning of ammeline production is also observed in the second“reaction”region as noted by its detection in the HPLC analysis of the residue at250◦C(Table1).Al-though CY A and ammelide are most likely produced via parallel processes,ammeline could be produced either by ammination of ammelide,Eq.(11),or as suggested by Ha-effele et al.[20],by the direct reaction between HNCO and remaining urea,Eq.(12),or some modification thereof.No other species is detected in our analysis that could serve as a precursor to ammeline.However,at all temperatures up to350◦C,the mass amounts of ammelide exceed ammeline in the residue(Table1).Although this is not proof of a lin-ear process,it has been used as supporting evidence[24]. As previously mentioned,however,ammination reactions are only likely to occur at elevated pressures[18]or tem-peratures[19].The intermediate for Eq.(12)is most likely biuret.Ammeline production:ammelide(s)+NH3(g)→ammeline(s)+H2O(g)(11)or2HNCO(g)cyanic acid+H2N–CO–NH2(m)urea→ammeline(s)+2H2O(g)(12) If this occurs,Eq.(12)can be written in the following com-bined form assuming the previous occurrence of Eq.(2). This is a very likely scenario:4H2N–CO–NH–CO–NH2(m)biuret+HNCO(g)cyanic acid→ammeline(s)+2H2O(g)(12a) The evolution of gases begins to visibly slow as a white precipitate forms at temperatures exceeding210◦C.Con-version of the melt into a“sticky”solid matrix is complete at225◦C,and CY A becomes the major component of the residue.Analysis of trapped off-gases for[NH4+]ion col-lected between210and225◦C,gives8500ppm(Table3). This is an indication that decomposition of urea and biuret4Eq.(12a)is very similar to Eqs.(3)and(7).The latter two reactions are relatively slow and most likely continue to be so even at elevated temperatures.Eq.(12a)is probably even slower than either Eq.(3) or Eq.(7)at all temperatures,and is likely the reason ammeline is not observed until this point.(Biuret+HNCO reaction rates:Eq.(3) producing CY A>Eq.(7)producing ammelide>Eq.(12a)producing ammeline.)138P.M.Schaber et al./Thermochimica Acta424(2004)131–142Table4HPLC Mass Table(biuret pyrolysis)aTemperature(◦C)Mass(g)Biuret(g)CY A(g)Ammelide(g)Ammeline(g)Melamine(g)Total(%)recovery 22565.028.634.5 2.860.26–101.826051.0 6.6340.8 3.830.36–101.227539.00.0835.5 3.470.35–101.0a These data were calculated based on the results obtained from HPLC and TGA analysis assuming100.0g of urea initially present.continue in this temperature range.The continued produc-tion of CY A and ammelide,Eqs.(9)and(10),are also ex-pected to contribute to the NH3(g)production as well.Analysis for[NH4+]ion gives a maximum in the residueat225◦C.This represents a substantial increase from thevalue observed at190◦C(Table2).Accumulation of[NH4+]is a direct consequence of system precipitation.As the ureamelt is transformed into a solid matrix,the rate at whichNH3(g)and HNCO(g)can diffuse from the system is di-minished.These species are either trapped in the solid ma-trix,or interact with each other,Eq.(13),and exist in theresidue in ionic form.The fact that ionic formation occurs issupported by the[NH4+]analysis of the residue from thesestudies,and by the MS data collected by Carp[15].Just asdecomposition species are“tied up”in ionic formAmmonium cyanate production:NH3(g)ammonia +HNCO(g)cyanic acid→NH4+NCO−(s)ammonium cyanate(13)in the matrix,5it is also likely that the remaining urea and biuret are likewise converted to ionic cyanurates via inter-action with CYA,Eqs.(14)and(15).Urea cyanurate production:H2N–CO–NH2(m)urea+CY A(s)→H2N–CO–NH3+CY A−(s)urea cyanurate(14)Biuret cyanurate production:H2N–CO–NH–CO–NH2(m)biuret+CYA(s)→H2N–CO–NH–CO–NH3+CY A−(s)biuret cyanurate(15)The narrow plateau region observed near225◦C in the urea TGA corresponds very well to a more demonstrable one exhibited by biuret at approximately the same temper-ature(Figs.2and4,respectively).In the biuret system, the residue is primarily composed of biuret and CY A,with lesser amounts of ammelide and ammeline(Table4).Since all the product species are thermally stable at225◦C,a plateau region is observed in both the urea and biuret TGA at this temperature.FT-IR data collected on the off-gases resulting from the urea residue at this temperature illustrates that gaseous emissions have greatly decreased.Only a small5Eq.(13)is in essence the reverse of the latter portion of Eq.(1).amount of NH3(g),HNCO(g)and HNCO salt speciesare emitted from the residue matrix.Emission thereforecontinues,but at a much reduced rate[12].Melamine is positively identified for thefirst time at250◦C6(Table1).This represents a departure from thetemperature and level at which this product isfirst observedunder TGA conditions(350◦C,and in substantially smalleramounts)[2].The direct ammination of ammeline,Eq.(16),is one possible route to melamine.Once again,althoughthis may be the simplest conceptual route,it is one likelyonly to be observed atMelamine production:ammeline(s)+NH3(g)→melamine(s)+H2O(g)(16) elevated pressures[18]or temperatures[19].However,openreaction vessel conditions as applied here may produce lo-calized temperature conditions favoring this process to alimited extent.Another possibility is cyanamide(H2NC≡N) trimerization[10],Eq.(17).Although cyanamide has notbeen isolated from urea decomposition under normal3H2NC≡N(g)cyanamide→melamine(s)(17)pressure[11],it could be produced via an alternate decom-position route associated with urea dehydration,Eq.(18), or by ammination of cyanic acid[10],possibly within the solid matrix,Eqs.(19)and(19a),and behave as a very reac-tive intermediate at these temperatures.The small amounts of melamine observed may be reflective of small Cyanamide production:H2N–CO–NH2(m)urea→H2O(g)+H2NC≡N(g)cyanamide(18)6This is somewhat of an anomaly since melamine is reported to melt with accompanying sublimation below250◦C.It is therefore necessary to assume that if melamine forms,it sublimes simultaneously as it is being produced.The sublimation of melamine may therefore also contribute to the mass loss observed between225and250◦C in the TGA(Fig.2). Accumulation of measurable amounts of melamine,result from production that is kinetically faster that sublimation.It should also be noted that melamine is observed at250,275,and350◦C,but not at320◦C(Table1). This would suggest different mechanisms for its production in different temperature ranges.At lower temperatures(below300◦C),Eq.(17),or some other unidentified reaction,results in production of melamine.At higher temperatures,Eq.(16)is expected to predominate.。
异丙苯过氧化物的热解反应及联枯的生成
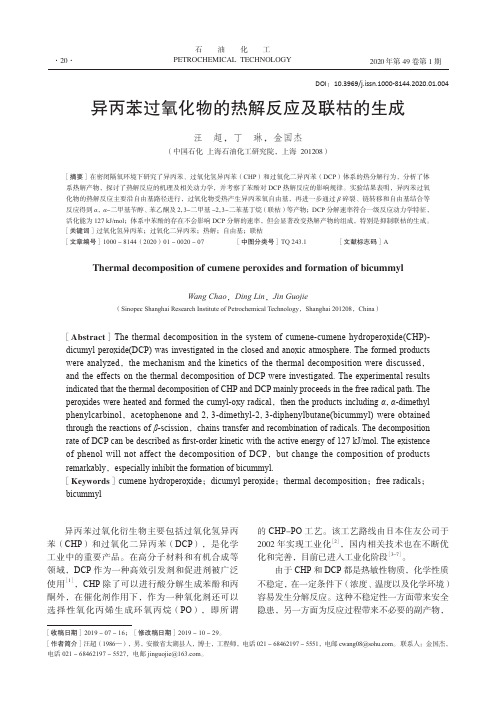
异丙苯过氧化物的热解反应及联枯的生成汪 超,丁 琳,金国杰(中国石化 上海石油化工研究院,上海 201208)[摘要]在密闭隔氧环境下研究了异丙苯、过氧化氢异丙苯(CHP )和过氧化二异丙苯(DCP )体系的热分解行为,分析了体系热解产物,探讨了热解反应的机理及相关动力学,并考察了苯酚对DCP 热解反应的影响规律。
实验结果表明,异丙苯过氧化物的热解反应主要沿自由基路径进行,过氧化物受热产生异丙苯氧自由基,再进一步通过β碎裂、链转移和自由基结合等反应得到α,α-二甲基苄醇、苯乙酮及2,3-二甲基-2,3-二苯基丁烷(联枯)等产物;DCP 分解速率符合一级反应动力学特征,活化能为127 kJ/mol ;体系中苯酚的存在不会影响DCP 分解的速率,但会显著改变热解产物的组成,特别是抑制联枯的生成。
[关键词]过氧化氢异丙苯;过氧化二异丙苯;热解;自由基;联枯[文章编号]1000-8144(2020)01-0020-07 [中图分类号]TQ 243.1 [文献标志码]AThermal decomposition of cumene peroxides and formation of bicummylWang Chao ,Ding Lin ,Jin Guojie(Sinopec Shanghai Research Institute of Petrochemical Technology ,Shanghai 201208,China )[Abstract ]The thermal decomposition in the system of cumene-cumene hydroperoxide(CHP)- dicumyl peroxide(DCP) was investigated in the closed and anoxic atmosphere. The formed products were analyzed ,the mechanism and the kinetics of the thermal decomposition were discussed ,and the effects on the thermal decomposition of DCP were investigated. The experimental results indicated that the thermal decomposition of CHP and DCP mainly proceeds in the free radical path. Theperoxides were heated and formed the cumyl-oxy radical ,then the products including α,α-dimethyl phenylcarbinol ,acetophenone and 2,3-dimethyl-2,3-diphenylbutane(bicummyl) were obtained through the reactions of β-scission ,chains transfer and recombination of radicals. The decomposition rate of DCP can be described as first-order kinetic with the active energy of 127 kJ/mol. The existence of phenol will not affect the decomposition of DCP ,but change the composition of products remarkably ,especially inhibit the formation of bicummyl.[Keywords ]cumene hydroperoxide ;dicumyl peroxide ;thermal decomposition ;free radicals ;bicummylDOI :10.3969/j.issn.1000-8144.2020.01.004[收稿日期]2019-07-16;[修改稿日期]2019-10-29。
2题名+单位英文名
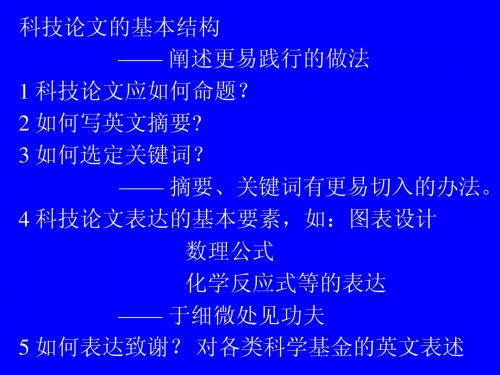
当有介词by时, 应保留investigation 之类 的词。
Investigation of phase behavior of polymer blends by thermal methods. A study of rate constants for two-phase electron-transfer reaction by A.C. impedance → A.C. impedance study of rate constants for two-phase electron-transfer reaction
The synthesis of a novel alcoholsoluble polyamide resin → Synthesis of a novel alcoholsoluble polyamide resin
• Avoid nonquantitative , meaningless words like “rapid” and “new”
“Speciation”(鉴别 分类)含义已经包括物质 的 各 种 化 学 形 态 、 形 态 分 析 。 “Chemical”、“Analysis”应删去。
举例
在三个升温速率下用热天平研究 煤的热解及其反应动力学 → 煤的热解及其反应动力学 PES/PC 共混体系的研究 → PES/PC 共混体系的力学性能; PES/PC 共混体系的相容性与力学性能
• In most cases, omit “the” at beginning of the title
The microstructure of microcrystalline cellulose → Microsபைடு நூலகம்ructure of microcrystalline cellulose
苯并恶嗪酚醛环氧豆油三元聚合体系的热分解行为研究徐丽
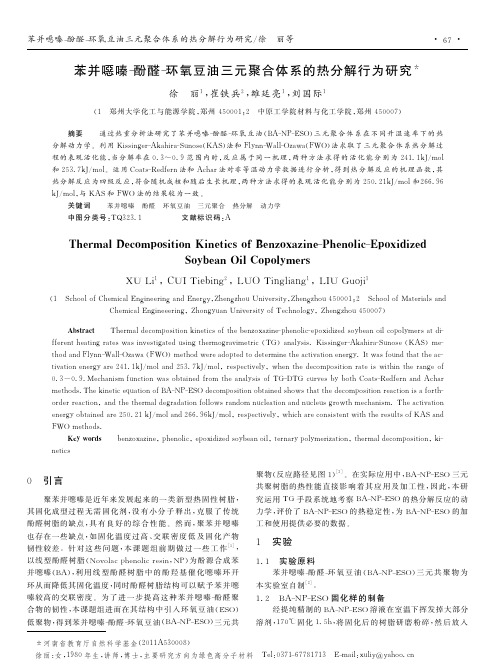
(1 School of Chemical Engineering and Energy,Zhengzhou University,Zhengzhou 450001;2 School of Materials and Chemical Engineeering,Zhongyuan University of Technology,Zhengzhou 450007)
苯 并 噁 嗪 -酚 醛 -环 氧 豆 油 三 元 聚 合 体 系 的 热 分 解 行 为 研 究/徐 丽 等
· 67 ·
苯 并 噁 嗪 -酚 醛 -环 氧 豆 油 三 元 聚 合 体 系 的 热 分 解 行 为 研 究 *
徐 丽1,崔 铁 兵2,雒 廷 亮1,刘 国 际1
(1 郑州大学化工与能源学院,郑州 450001;2 中原工学院材料与化工学院,郑州 450007)
摘要 通过热重分析法研究了苯并噁嗪-酚醛-环 氧 豆 油 (BA-NP-ESO)三 元 聚 合 体 系 在 不 同 升 温 速 率 下 的 热 分解动力学。利用 Kissinger-Akahira-Sunose(KAS)法和 Flynn-Wall-Ozawa(FWO)法 求 取 了 三 元 聚 合 体 系 热 分 解 过 程的表观活化能,当分解率在0.3~0.9 范 围 内 时,反 应 属 于 同 一 机 理,两 种 方 法 求 得 的 活 化 能 分 别 为 241.1kJ/mol 和253.7kJ/mol。运用 Coats-Redfern法和 Achar法对非等温动力学数 据 进 行 分 析,得 到 热 分 解 反 应 的 机 理 函 数,其 热分解反应为四级反应,符合随机成核和随后生长机理,两种方法求得的 表 观 活 化 能 分 别 为 250.21kJ/mol和266.96 kJ/mol,与 KAS和 FWO 法的结果较为一致。
有机硅改性形状记忆水性聚氨酯的制备

有机硅改性形状记忆水性聚氨酯的制备官慧;艾娇艳;卢子铿;卢杨陆;林洁明【摘要】将形状记忆水性聚氨酯用不同的硅烷偶联剂和端羟基硅油进行改性,并研究其改性前后的性能.结果表明:当硅烷偶联剂的质量分数为1%时,材料具有良好的力学性能、形状记忆性能和耐水性能;改性后,材料的形状固定率均为100%,形状回复率为96%以上;经硅烷偶联剂KH570改性的形状记忆水性聚氨酯的拉伸强度为49.96 MPa,较未改性体系提高了18.42%;端羟基硅油改性使材料质量损失50%的温度从370.21 ℃提高到378.13 ℃.%The shape-memory waterborne polyurethane(PU)was modified with different silicane coupling agents and hydroxyl-terminated silicone oil and whose performances before and after modification were tested. The results show that the material performs excellently in mechanical properties,shape memory and water resis-tance when the mass fraction of silicane coupling agent is 1%. The shape fixation rate is 100%,the shape reco-very rate is more than 96% after modification. The tensile strength of shape-memory waterborne PU modified by KH570 reaches 49.96 MPa,18.42% higher than that of unmodified system. The 50% weight loss temperature of the material modified by hydroxyl-terminated silicone oil is increased from 370.21 to 378.13 ℃ .【期刊名称】《合成树脂及塑料》【年(卷),期】2018(035)001【总页数】4页(P20-22,29)【关键词】水性聚氨酯;有机硅;形状记忆;改性【作者】官慧;艾娇艳;卢子铿;卢杨陆;林洁明【作者单位】广东工业大学材料与能源学院,广东省广州市 510006;广东工业大学材料与能源学院,广东省广州市 510006;广东工业大学材料与能源学院,广东省广州市 510006;广东工业大学材料与能源学院,广东省广州市 510006;广东工业大学材料与能源学院,广东省广州市 510006【正文语种】中文【中图分类】TQ323.8形状记忆水性聚氨酯在合成的过程中以水代替溶剂,减少了有机溶剂的挥发,符合当今环保要求[1-4]。
不饱和聚酯树脂的聚合及力学特性

在显微镜下对聚合产物形貌的观察,发现随着过氧化甲乙酮的加入量增大,聚合产物内的产生的气泡也越多,使其力学强度变弱. 研
究表明过氧化甲乙酮的加入量过大或过小会对聚合产物的力学特性产生不利的影响. 图 3,表 1,参 10.
关键词:不饱和聚酯树脂;聚合;固化剂;力学特性
中图分类号:TQ323.4+2;TQ 177.1
第 24 卷第 2 期 2009 年 6 月
湖南科技大学学报(自然科学版) Journal of Hunan University of Science & Technology(Natural Science Edition)
Vol.24 No.2 Jun. 2009
不饱和聚酯树脂的聚合及力学特性
(a)0.5%MEKP
(b)1.0%MEKP
(c)2.0%MEKP
图 3 加入不同量 MEKP 的试样镜下的形貌(×100)
Fig.3 The surface of specimen of MEKP at optics microscope (×100)
5 结论
在 UPR 与填料总量的比例为 7∶13 情况下,改变 MEKP 的加入量,对聚合产物的力学性质会有影响, MEKP 质量分数较小时 (为 0.5%~1.0%时),对 UPR 聚合的引发量不够,形成的体型聚合物的分子量不够 高,导致其力学性质不好. MEKP 质量分数在 1%左右 时抗弯强度和抗压强度都达得到了一个极大值.当 MEKP 的质量分数继续增大至 1.0%~2.0%时,固化反 应速度太快,导致缩ength/MPa
酚醛树脂(phenolic resin)

酚醛树脂(phenolic resin)1. introduction:Phenolic resin is the world's first found and thermosetting synthetic resin industry, as raw materials, synthesis process and convenient, has the mechanical strength and good heat resistance, has excellent mechanical properties and electrical properties, has the characteristics of high temperature creep resistance heat resistance, flame retardant, it is still widely used in plastics, friction material, adhesive, coating, casting resin, foam and other fields. However, with the rapid development of industrial economy, more and more requirements for the properties of phenolic resin have been put forward. The reaction mechanism, structure and properties of phenolic resin are still deeply studied. Many achievements have been made in toughening, reinforcing and modifying of phenolic resin. A variety of phenolic composite materials have also been listed as human engineering plastics series. Phenolic hydroxyl groups and methylene groups in phenolic resin structure are easily oxidized, so that their heat resistance is affected, and phenolic resin is brittle after curing. Therefore, until now, how to improve phenolic resin and apply it to industry is still an important topic.2. technology development:The development of bakelite, after all to the modification of phenolic resin modified phenolic resin, now mainly in the following aspects: 1. using natural plant oil modified phenolic resin; 2. using thermal plastic resin modified phenolic resin;3. low molecular chemical modified phenolic resin; modifiedphenolic resin 4. thermosetting resin; 5. rubber modified phenolic resin; 6. nano material toughened phenolic resin; 7. thermotropic liquid crystal toughened phenolic resin.2.1 natural vegetable oil modified phenolic resinThe natural plant oil modified phenolic resin is chemically modified, flexible long chain molecular structure in vegetable oil containing double bond or conjugated triple bond, by double or triple bond phenolic resin synthesized in the reaction process, the flexible long chain into rigid phenolic molecule chain, play in the role of toughening, thereby improving the phenolic resin brittle.2.1.1 cashew nut shell oil modified phenolic resinCashew nut shell oil is a by-product of cashew processing, and it is a very rich biological resource. Compared with phenol, cashew nut shell oil is much cheaper. From the structure of main components can be seen, the characteristics of cashew oil both phenolic compounds, aliphatic compounds and flexible, with the modified phenolic resin, heat resistance and toughness were significantly improved and modified products used for friction materials, friction performance, flexible film formed on the surface of carbonization process of friction easy to fall off, the friction and heat state of the material surface evenly, ensure stable friction properties. A cashew oil modified phenolic resin was synthesized by Novalac Liu Xuemei, the middle shell oil content is continuous, notched impact strength and flexural strength of cashew oil modified phenolic resins and molding materials were increased by 10.7% and 14.8%. Thisis because the long alkane chain structure of cashew shell oil in existence, the equivalent in phenolic resin matrix are introduced in the flexible chain, to the toughening effect of phenolic resin, so as to increase the notched impact strength of molding materials prepared and bending strength.2.1.2 tung oil modified phenolic resinWhen tung oil reacted with phenol, tung oil became positive ion under the action of acid catalyst, and then reacted with phenol instead of phenol. When the ratio of phenol / tung oil is greater than 6, the interpenetrating network of phenolic resin modified by tung oil and tung oil is obtained, thus improving the toughness of phenolic resin. Shang Shibin with tung oil, bismaleimide maleic anhydride, reaction with phenolic resin, prepared Eleostearic Acid Anhydride imide phenolic resin by thermal analysis method of tung oil anhydride imide resin at 170 degrees bake for 4 h, the measured temperature index of up to 165.8 DEG C, indicating a good heat-resistant resin. Li Chao of tung oil / phenolic resin and nano SiO2 / 2 phenolic / tung oil modified phenolic resin were studied. The results show that the decomposition temperature in 420 degrees Celsius above their initial heat, nano SiO2 / tung oil / phenolaldehyde system heat resistance is better than that of phenolic / tung oil, and 600 C, the former than the latter in each a focus on the loss of heat temperature has increased by 30 to 50 DEG C. A friction material made of this tung oil modified phenolic resin,Not only the friction coefficient of low temperature (100~200 DEG C) remains at 0.45 ~ 0.51, but also the friction coefficient at high temperature (200~300 DEG C) is up to 0.38 ~ 0.51, andthe friction factor recovery performance is good, and can be maintained at more than 0.40.2.1.3 Catalpol modified phenolic resinThe phenolic resin modified by catalpa oil is a kind of resin which reacts the excess phenol with vegetable oil under acidic conditions, and then reacts with formaldehyde under alkaline condition to produce the resin. The principle is similar to that of Tung oil. It was found that the toughness and heat resistance of phenolic resin modified by catalpa oil were improved.2.1.4 linseed oil modified phenolic resinLinseed oil is eighteen carbon three acid esters of glycerol, 3 non conjugated double bonds in the molecule, in the presence of acid and linseed oil non conjugated three ene of nuclear phenol alkylation reaction, obtained linseed oil modified phenolic resin, improve the toughness of resin. Study on phenolic resin modified by linseed oil on Yuan Xinhua's show in 320 ~ 600 DEG C linseed oil modified phenolic resin was 53.11%, while the ordinary phenolic resin 73.51% weight loss; the peak temperature of thermal decomposition, common phenolic resin 400 ~ 425 and 540 ~ 600 DEG C, and linseed oil modified is 440 ~ 470 C and 600 to 660 DEG C. Linseed oil modified phenolic resin for testing wear properties and mechanical properties of friction material showed that the modified resin adhesion and toughness are improved, the friction coefficient of the material is large and stable, low wear rate, impact strength, hardness, can be used as matrix resin for high performance friction materials.2.2 thermoplastic resin modified phenolic resinThe blending of thermoplastic resin with phenolic resin is a simple and easy way to strengthen and toughen. Thermoplastic resins for strengthening and toughening include polyether, polyamide, polyvinyl alcohol and polyvinyl acetals.2.2.1 polyether modified phenolic resinGe Dongbiao et al modified phenolic resins with a series of different molecular weight polyethylene glycol (PEG) and active polyethers. The tensile strength and elongation at break of PEG modified phenolic resin begin to increase with the increase of molecular weight of PEG. When the molecular weight is 1000, the peak value is reached, and then decreases with the increase of molecular weight of PEG. For the same molecular weight PEG and active polyether, the tensile strength and elongation at break of the modified polyether are better than those of the corresponding PEG modification. Among them, the tensile strength and elongation of PEG1000 modified phenolic resin 11.546MPa and 2.358% respectively; the tensile strength and elongation of 1000 active polyether modified phenolic resin 19.032MPa and 3.779% respectively. In addition, the effect of toughening modification of active polyether is better than that of carboxyl terminated nitrile rubber. This is mainly because the hydroxyl of -NCO based active polyether resin reaction more easily and makes the introduction of flexible ether phenolic resin chain network system, and the reaction probability of hydroxy carboxyl and hydroxyl of PEG1000 resin and carboxyl terminated nitrile rubber in the very small, tend to be morephysical modification.2.2.2 polyamide modified phenolic resinPolyamide has good toughness and mechanical properties. It can be co cured with phenolic resin or form a partially interpenetrating network structure to improve mechanical properties of the resin. Gao Yuejing, with three yuan of nylon modified phenolic resin was found in modified phenolic resin system, nylon as dispersed phase, phenolic resin as continuous phase copolymerization of dispersion phase particle size distribution was narrow, the mechanical properties of plastic is much better than that of blends of plastic material. The impact strength of modified nylon resin modified by unmodified resin, blending three yuan nylon and copolymerization three yuan is 57 KJ/m2, 47.1 KJ/m2 and 112 KJ/m2 respectively. The properties of copolymer modified resins are best due to the formation of graft phase copolymers during the synthesis and curing of modified resins. In addition, the phenolic resin toughened with nylon 3 has obtained good results. The nylon 3 molecule contains amide groups which react with hydroxymethyl groups in the phenolic resin, thereby improving the impact toughness and adhesion of the phenolic resin, and maintaining the advantages of the phenolic resin. The impact strength of the resin before and after modification is 3.5 to 4.5KJ/m2 and 5~12 KJ/m2 respectively, and the tensile strength is 7 to 11MPa and 15 to 20MPa respectively.2.2.3 polyvinyl alcohol modified phenolic resinIn the curing process of phenolic resin, if the PVA is added, the hydroxyl groups on the polyvinyl alcohol will react with the hydroxymethyl group of the phenolic polymer to form the graft copolymer. Jiang Detang in the development of a new HF- I modified epoxy resin, adding polyvinyl alcohol modifier. The results show that the addition of modifier to improve resin adhesion, improve the brittleness of phenolic resin, reduce the curing rate, thereby reducing the molding pressure, the toughening effect of phenolic resin on. However, the amount of use should not be too high, otherwise it will affect its strength.2.2.4 polyvinyl butyral modified phenolic resinThe phenolic resin modified with polyvinyl alcohol acetal is a modified phenolic resin which is applied earlier in industry. Under heating conditions, hydroxyl groups in polyvinyl acetals can undergo dehydration reactions with hydroxyl groups in phenolic resins to form graft or block copolymers. Polyvinyl alcohol acetal can improve the brittleness of phenolic resin, improve adhesion and mechanical properties, and reduce molding pressure. However, because of the introduction of longer fat chains in the structure of phenolic resins, heat resistance is affected. In order to improve the heat resistance, the polyvinyl alcohol acetal with higher heat resistance is often used, and a certain proportion of organosilicon monomer is added.2.3 low molecular chemical modified phenolic resin2.3.1 dicarboxylic acid modified phenolic resinThe molecular weight of the polymer molecules can also be used to improve the brittleness of resole resin (DA4), adipic acid, suberic acid (DA6), sebacic acid (DA8) and twelve alkyl acid (DA10) can be used to improve the brittleness of resole resin.A study found that the elasticity of dicarboxylic acid and resole resin in curing process in the reaction of ester bond formation, and also can be formed in the dibasic acid ester bond of carboxyl and hydroxyl methyl reaction into phenolic resin in brittle tensile strength of modified resin, toughness and fracture elongation increased with the increase of DA8 content, but the content in per gram of resin DA8 is over 0.014mol the performance decreased. Indicating the incorporation of flexible groups containing dibasic acid can improve the brittleness of resin. The performance of the modified resin decreased after the content of DA8 exceeded 0.014mol, indicating that more DA8 would lead to a decrease in the properties of the resin, or an unreacted DA8 would become defective in phase separation. When the phenolic resin was modified with DA6 with the best molecular chain length, the tensile strength of the resin increased by 54% and the toughness increased by 64%.2.3.2 halogenated phenolic resin modified by cyanogenLinear phenolic resins can react with cyanogen halides to form phenolic three - triazine resins. Novolac resin first with cyanogen bromide and three yuan amine reaction of Novolac Cyanate Ester and Novolac Cyanate Ester in the heat under the action of the cyclization reaction of three, eventually formingthree phenolic cyanate resin mesh structure. Phenol three Benzoxazine Resin has the following characteristics: the solubility of melt viscosity and gel time length, thermal oxidative stability, long-term in a low boiling solvent high low, under different curing conditions of Tg can reach 399 DEG C, polyimide system elongation and fracture mechanics with high performance, low toxicity, low the flame retardancy and smoke rate. At the same time, the network structure of phenolic three triazine contains tenacity ether bond, so it has better toughness after modification.2.3.3 molybdenum modified phenolic resinMolybdenum phenolic resin (Mo2PF) is a modified phenolic resin in which the metal element molybdenum is chemically bonded to the main molecular chain of the phenolic resin by chemical reaction. Is the reaction by molybdate and phenol in the presence of catalyst into molybdate benzene ester, phenyl ester and molybdate addition reaction and condensation reaction with formaldehyde, generation of phenolic resin, after cooling is a green solid, with the increase of molybdenum content in the resin resin, the initial decomposition temperature rise. Liu Xiaohong et al made aluminum phenolic resin with aluminum modifier, phenol and formaldehyde as main raw materials. The metal molybdenum could enter the molecular chain of phenolic resin, and the great bonding O - MO - O bond could connect the benzene ring to improve the heat resistance. The thermal decomposition temperature of modified phenolic resin prepared by this method can reach 522 DEG C, and the weight loss is only 17.5% at 600 centigrade,Other properties are comparable to that of ordinary phenolic resins. In addition, the in situ polymerization method was used to improve the heat resistance, impact properties and tensile properties of the molybdenum phenolic resin /TiO2 nanocomposite by filling and modifying the surface treated nano TiO2. Zhang Shuangqing with phenol and formaldehyde in the first synthesis of hydroxymethyl phenol, and molybdic acid and hydroxymethyl by esterification reaction to obtain a new thermosetting phenolic resin and its carbon residue rate was 56.31%, the rate of 13 percentage points higher than the average carbon residue of thermosetting phenolic resin.2.3.4 boron modified phenolic resinThe modification of phenolic resin with boron compounds, the change of its structure, the formation of B - O bond with higher bond energy and the formation of boron - containing three - direction crosslink structure are one of the effective methods to improve its heat resistance. Che Jianfei by in situ synthesis method with TiO2 nanoparticles added to the boron phenolic resin, can significantly improve the heat resistance and toughness, when 5% TiO2 was added, the initial decomposition temperature is increased by about 150 degrees, the impact strength increased 231%. In addition, the processing technology of modified phenolic resin was also improved obviously. The introduction of boron is also beneficial to improve the flame retardancy of phenolic resin. Modified phenolic resin with boric acid and Aman Chang [16], the product still has a solid residue 75.3% in 700.8 DEG, 900 DEG C without decomposition residue is still above 64%, far higher than similar foreign products (700 C solid residue 63%). Theflexural strength, flexural modulus and impact strength of the composites obtained by Qiu Jun and other carbon fiber composite boron phenolic resins have been greatly improved. Tung oil, high performance double boron modified phenolic resin was synthesized by two steps such as Wang Manli, used it as a non asbestos braided brake belt has good friction performance of matrix resin, reached the level of similar foreign products.2.3.5 amine modified phenolic resinIs the main aromatic amine compounds and total phenol and formaldehyde condensation reaction in the presence of catalyst, introducing aromatic amine unit structure and good heat resistance in the structure of the phenolic resin, aromatic amines are commonly used melamine and melamine methylol compound and aniline. Amine modified phenolic resin, the heat resistance is improved, thermogravimetric analysis results show that aniline modified phenolic resin thermal decomposition temperature is 410 DEG C, melamine modified resin is 438 DEG, 380 DEG C than the pure phenolic resin to improve its heat resistance is high, reason is the introduction of a more stable heterocyclic the construction and curing resin crosslinking density increased. The prepared friction material has good friction property at high temperature, and it is a common modification method.2.3.6 silicone modified phenolic resinSilicone modified phenolic resin has high heat resistance, thermal stability, toughness and other excellent properties, the modified method is: the phenolic resin is reacted withorganosilicon compounds containing alkoxy groups, forming a three-dimensional network structure containing silicon oxygen bond, there is a competitive reaction from poly phenolic reaction process, so two kinds of reactivity is the key to the success of the modified. Allyl phenolic resin reacted with organosilicon compound to form organosilicon modified phenolic resin with excellent heat resistance. The tribological properties of the friction material modified with silicone resin are stable and the wear rate is low. The friction coefficient varies little (=0.136 ~ 0.140) at the temperature range of 100 ~ 350 DEG CPhenolic resin modified by 2.3.7 aromaticsThe modified aromatic hydrocarbons are toluene, xylene, substituted benzene, naphthalene and so on. The modified principle is introduced so that the stability of the aromatic molecules increased, increased rigidity, thereby improving the heat resistance modification methods were as follows: the main aromatic hydrocarbons (Ar) reacts with formaldehyde to produce aromatic alcohols, and then generate the phenol and formaldehyde resin. Aromatic hydrocarbon, phenol and formaldehyde react simultaneously to form resin. The heat resistance of the modifier depends on the structure of the aromatic compound. The 53 Research Institute of ordnance industry has achieved satisfactory results by using the tower substrate (aromatic compound) extracted from xylene to modify phenolic resin. The modified route using first methods, modified phenolic thermal decomposition temperature above 450 degrees, thanThe phenolic resin is higher than 50 DEG C, and the weight loss rate at high temperature increases slowly,Suitable for high temperature use, the friction material produced has good friction performance, high temperature coefficient of friction is high and stable, low wear rate.2.4 thermosetting resin modified phenolic resin2.4.1 polyimide modified phenolic resinPolyimide is composed of two aromatic amines and two anhydrides. It has excellent heat resistance and flame retardancy, and can remarkably improve the heat resistance of phenolic resin. Yan Yehai and so on were synthesized bismaleimide modified phenolic resin I, the cured resin has outstanding heat resistance, the thermal deformation temperature (HDT) is 273 DEG C, and the glass temperature is 288 DEG C. The structure content of high phenyl ring and high cross linking density are the structural basis for the excellent heat resistance of cured resins. Phenolic resin II was obtained by blending resin I with appropriate high temperature curing agent. Resin II with deformation temperature and glass transition temperature of the bending performance of more excellent and more heat than resin I, use this source in high temperature curing agent to improve the crosslinking density of the cured resin, so as to ensure the heat resistance of the cured resin. Resin I and II with glass cloth composite materials with excellent mechanical properties and heat resistance based at 300 DEG C based on the flexural strength and modulus of I resin composite material retention rate is respectively 65% and 78%, the II phenolicresin composites flexural strength and modulus retention rate of up to 73% and 83% respectively.2.4.2 polysulfone modified phenolic resinPolysulfone has excellent heat resistance, outstanding creep resistance and dimensional stability, excellent electrical insulation and other characteristics. It has become a comprehensive engineering plastics, and occupies an important position in plastic varieties. Dong Ruiling, Qi Shuhua and others found that polysulfone modified phenolic resin, polysulfone in the form of grafting and blending exist simultaneously. The modified phenolic resin glass fiber reinforced plastic toughness is improved, the impact strength increased from 200KJ/m2 to 283KJ/m2, the tensile strength of 960MPa, the dielectric strength reached 20KV/mm, Martin heat resistance temperature more than 310 DEG C. The glass fiber reinforced mould plastics made of this kind of polysulfone modified phenolic resin have been successfully applied to aerospace products.Phenolic resin modified with 2.4.3 maleimide polymerIn order to improve the toughness and heat resistance of phenolic resin, maleimide resin was used as modifier. The modified resin had high toughness and heat resistance, and the modification effect was obvious. Novolac resin with maleimide groups (PMF) is composed of phenol and N- mixture and 4 hydroxy phenol formaldehyde polyimide under acid catalysis reaction and its curing, mainly through maleimide group addition polymerization. This system greatly improved the heatresistance of phenolic resin and improved the thermal stability of the resin in the higher temperature region, but the toughness was not improved. Someone uses a new addition curing system to improve the toughness of resin system, this system is composed of PMF resin and novolac resin containing allyl group (PAF) according to the different ratio of mixed into, in the reaction with allyl blends.With the increase of the content of propyl phenol, the crosslinking density of the cured matrix decreases, the toughness increases, and the mechanical properties increase. Under the curing conditions, the optimum performance is obtained when the equivalence ratio of allyl and maleimide is 1 to 3.2.4.4 epoxy modified phenolic resinPhenolic hydroxyl groups and hydroxymethyl groups on phenolic resins can react with epoxy groups and hydroxyl groups in epoxy resins to form an cross-linked structure, which reduces the crosslinking density of the cured products and increases the flexibility of the molecules. The study of Jiang Detang and others showed that the brittleness of phenolic resin was improved and the mechanical properties were obviously improved after adding epoxy resin. When the equivalence ratio between epoxy and phenolic resin was 1 to 3, two diglycidyl ether bisphenol A epoxy modified phenolic resin was obtained with the largest KIC (0.85MPa, m1/2). The properties of the composites are also considerably improved than those of DDS curable materials. When the content of novolac resin in thecross-linked network structure increases, the Tg of themodified resin decreases and the toughness increases. In addition, with the increase of linear phenolic resin content, the flame retardancy of the modified resin will increase significantly. Therefore,We can obtain a dense system with excellent mechanical properties and flame retardancy by controlling the content of novolac resin.2.5 rubber modified phenolic resinMany large molecules such as Ding Jing, styrene butadiene rubber and natural rubber were used to toughen phenolic resin. The rubber toughened phenolic resin is a kind of physical mixing modification, but there are different degrees of graft or block copolymerization in the course of curing. Li Xinming et al [17 study found that without nitrile rubber, the impact strength of pure phenolic resin was 5.43 KJ/m2, adding 1% nitrile rubber, the impact strength reached 8.60KJ/m2, an increase of about 58.4%; nitrile rubber with 2%, the impact strength of phenolic resin reached 11.49 KJ /m2, increased by about 116%, improve the the amplitude is the other modification methods can not be compared. With the increase of NBR dosage, the impact strength of phenolic resin is further improved, but the amplitude increases. In addition to the nitrile rubber, rubber containing active functional groups such as epoxy based liquid butadiene rubber, acrylic acid carboxylated nitrile rubber and epoxy adduct can also toughened phenolic resin, and the toughening effect, improved heat resistance. Especially in the liquid rubber toughening system, because the liquid rubber is easy to form the island structure, the form structure which improvedthe impact strength of the composite hardness, heat resistance and little influence on the materials, is an ideal toughening system.Phenolic resin toughened with 2.6 nanometer materialThere are two main methods: 1 nanoparticles toughened polymer nanoparticles and polymer blends; using nano material intercalation method, namely the first polymer monomer is dispersed into layered silicate layers, then in situ polymerization. The polymerization of the released heat can be put into the stripping layer structure of silicate structure unit thickness 1nm width and length of about 10nm, which are uniformly dispersed in the phenolic resin, composite resin and stripping unit structure on the nanometer scale. The toughened phenolic resin has better toughness and thermal stability than the conventional toughened phenolic resin.2.7 thermotropic liquid crystal toughened phenolic resinToughening of thermosetting resins with thermotropic liquid crystals improves toughness and toughness while maintaining heat resistance and stiffness of the resin. Liquid crystalline macromolecules contain a large number of rigid mesogenic units and a certain amount of flexible spacers, and their structural characteristics determine their excellent properties. The presence of a small amount of thermotropic liquid crystalline polymer fibrils can prevent cracks and improve the toughness of the brittle matrix without decreasing the heat resistance and stiffness of the material. Compared with thermoplastics, the same toughening effect can be obtained by using only 25%- 30% of thermoplastic3. conclusionAt present, the toughening modification of phenolic resin on maturity, there have been many modified phenolic resin with high performance, such as excellent mechanical properties, high temperature resistance, electrical insulation, flame retardant, low smoke and low toxicity and mechanical processing of new phenolic resin. It can be predicted that with the continuous development of aviation, aerospace, automobile and electronics industry, study on modified phenolic resin constantly, will make "phenolic resin old" further development, play a greater role in more and more fields.Reference[1]., Min, Ho, Choi, Ho, Yun, Byun, In, Jae, Chung., Theeffect, of, chain, length, of, flexible, diacid, on, Morphologyand, mechanical, property, of,, modified, phenolicresin.Polymer, 2002,43 (): 4437 ~[2]. He J, Raghavan D, Hoffman D, Hunston D. Theinfluence of elastomer concentration on toughness in dispersions containing preformed acrylic elastomeric particles in an epoxy matrix?.Polymer1999,40 (8): 1923 ~ 1933.[3]., Reghunadhan, N, C, P., Advances, in, cure, phenolic, resins., Progress, addition-, in, Polymer, Science.2004,29 (5): 401 ~。
半核壳 PS@Ag/Fe/Ag 阵列的制备与 SERS 性能研究

半核壳 PS@Ag/Fe/Ag 阵列的制备与 SERS 性能研究华中;孙欢欢;张梦宁;刘毛毛;陈雷;韩无双;张永军【摘要】表面增强拉曼散射( SERS)光谱已广泛应用在生命科学、化学、医学等诸多领域.设计了一种二维有序的半核壳SERS活性基底.利用自组装技术制备聚苯乙烯( PS)球核,通过磁控溅射系统在其表面包覆Ag/Fe/Ag三层壳,改变Fe的溅射时间,制成不同厚度铁磁性层的半核壳PS@Ag/Fe/Ag阵列.采用532 nm激发波长,选择对巯基苯胺作为探针分子研究SERS基底的活性,其增强因子可达到107.SERS的增强主要来源于物理增强(即电磁场增强),实现基底的高灵敏度检测和重现性.铁磁性层的引入对PS@Ag/Fe/Ag阵列的SERS增强具有较好的调控作用.%Surface-enhanced Raman scattering ( SERS ) spectroscopy has been widely used in life science , chemistry ,medicine and many other areas .In this paper , we presented a two-dimensional ordered polystyrene ( PS) microsphere array by self-assembly technology and used magnetron sputtering system to prepare semi-nanoshell array of Ag/Fe/Ag on the PS array .By changing sputtering time of magnetic layer Fe ,we prepared the different thicknesses of core-shell SERS active substrates .We applied an excitation wavelength of 532 nm and used P-aminothiophenol as a probe molecule to study SERS property and enhancement factor can reach 107 .【期刊名称】《吉林师范大学学报(自然科学版)》【年(卷),期】2016(037)003【总页数】4页(P23-26)【关键词】表面增强拉曼散射;半核壳;SERS基底;磁性层【作者】华中;孙欢欢;张梦宁;刘毛毛;陈雷;韩无双;张永军【作者单位】吉林师范大学物理学院,吉林四平136000;吉林师范大学物理学院,吉林四平136000;吉林师范大学物理学院,吉林四平136000;吉林师范大学物理学院,吉林四平136000;吉林师范大学化学学院,吉林四平136000;吉林师范大学物理学院,吉林四平136000;吉林师范大学物理学院,吉林四平136000【正文语种】中文【中图分类】O657.37自1974年首次发现表面增强拉曼散射(SERS)效应以来,由于其具灵敏度高、无水性干扰、对样品无损伤等优点而被应用于材料学、化学、生物医学等许多领域[1].SERS能被广泛应用的关键就是其着力于SERS活性基底的发展.至今,不同材料和各种结构形状的SERS基底已被研究[2-3].对比其他材料,银(Ag)是最适合用于SERS研究的材料.Nie和Kneipp小组已经报道Ag基底可产生较大的增强效果[4-5],并且这种Ag基底能够用于单分子检测[6]、蛋白质免疫检测[3,7]、DNA检测[8]等.目前单纯的Ag研究颇多,然而许多过渡金属也可以产生较弱的SERS信号,将贵金属(Au、Ag、Cu等)和过渡金属(Fe、Co等)结合形成新型SERS基底研究并不多见[9-10].到目前为止,公认的SERS增强机理主要有两种:电磁场增强和化学增强,电磁场增强的贡献源于表面等离子体共振,即金属中的自由电子在光电场的激发下发生集体性振荡;而化学增强主要贡献在于吸附的探针分子与样品基底表面会发生电荷转移,以致整个样品体系的极化率发生明显的变化从而影响SERS信号[11-12].本文通过磁控溅射系统在PS球核阵列表面分别溅射Ag、Fe、Ag材料,其中衬底层Ag和覆盖层Ag溅射厚度均为20 nm,改变Fe壳溅射的时间,以制成不同厚度的半核壳PS@Ag/Fe/Ag材料.利用振动样品磁强计(VSM)测量样品磁性,通过扫描电子显微镜(SEM)观察样品的形貌,采用拉曼光谱仪来测量样品的SERS信号,选择波长为532 nm的Ar+激发线激发.PS@Ag/Fe/Ag基底最佳增强因子可达到107.由于Fe溅射厚度为8 nm的样品球壳间隙可以产生较多的局域等离子体热点,这些热点分布在球壳之间的连接处,其电磁场高于周围其他位置的电磁场,因此可以得到较强的SERS信号.对巯基苯胺(PATP)、十二烷基硫酸钠和乙醇购自于Sigma-Aldrich公司;用尺寸为200 nm的单分散聚苯乙烯胶体颗粒(PS)来组装模板;纯度为99.99% Ag靶和Fe靶均购自于北京天齐高级材料有限公司;实验用水为去离子水,电阻率为18.0 MΩ·cm.半核壳PS@Ag/Fe/Ag多层膜是在磁控溅射系统中制备的,溅射前的背景真空度为2.0×10-4 Pa,靶材距离其硅晶片20 cm.高纯度的氩气(99.99%)作为工作气体,工作压强为0.6 Pa.Ag和Fe靶的溅射功率分别为21.6 W和70 W.样品的形貌用扫描电子显微镜(SEM)表征.扫描电子显微镜(SEM)的型号是JSM7800F,其高分辨率高达1.5 nm,加速电压为5.0 kV.利用振动样品磁强计(VSM)对磁性进行表征,型号是M-7407,对样品所加的磁场为104 Oe.拉曼光谱是在Renishaw2000显微拉曼光谱仪上采集的,波长为532 nm的激发线是由Ar+激光器产生的.半核壳PS@Ag/Fe/Ag材料的制备流程如图1所示.首先利用自组装技术[13],在Si基底上制备单层有序的直径为200 nm的PS微球阵列;然后通过Ag靶直流溅射和Fe靶射频溅射在PS阵列上依次溅射Ag、Fe、Ag材料,衬底层Ag和覆盖层Ag厚度相同,均为20 nm,改变Fe溅射的时间,最终得到不同铁磁性层厚度的半核壳PS@Ag(20 nm)/Fe(t nm)/Ag(20 nm)多层膜结构阵列.图2给出了半核壳结构的SEM图,(A)—(E)图的Fe溅射厚度分别为2 nm、4nm、8 nm、16 nm和32 nm,(F)为密排的单层PS阵列.由图2(F)可以看出,组装在Si基底上的粒子单分散性好,呈六角密堆形图案.对比(F)可知,从(A)到(E),沉积在金属膜的PS阵列周期性保持完好,随着Fe溅射厚度的增加,球壳的表面粗糙度呈现出先增大后减小的趋势.这是由于随着Fe溅射时间的延长,半核壳逐渐变厚,势垒在粒子间的迁移过程中会随之增强,同时粒子间的化学势能差增大,跃迁的激活能减小,导致半核壳薄膜间的缺陷更容易被填充.当球壳的顶部生长趋势与缺陷的被填充情况相平衡时,薄膜的粗糙度将会降低.图3给出了不同厚度的半核壳PS@Ag/Fe/Ag的磁滞回线图.随着Fe溅射厚度的增加,磁化强度呈现先增大后减小的趋势,Fe层溅射厚度为8 nm时磁化强度达到最大,但都表现出明显的弱磁性.鉴于PATP 探针分子中的巯基中的S原子和金属能形成稳定的化学键,胺基官能团与金属可以发生相互作用,因此我们选用10-3 mol/L的PATP溶液为探针分子对PS@Ag/Fe/Ag基底进行SERS测量.图4为Fe溅射厚度不同的半核壳PS@Ag/Fe/Ag活性基底对浓度为10-3 mol/L的PATP溶液测试的拉曼光谱图,插图给出了在峰位1 430 cm-1处,不同SERS强度随着Fe溅射厚度的变化关系图.从插图明显看出,SERS强度与Fe壳的厚度呈非线性关系,SERS强度随Fe溅射厚度的增加呈现增强后减弱的趋势.当Fe壳溅射厚度为8 nm时,SERS信号最大.这主要是由于随着Fe溅射厚度的增大,磁性层逐渐变得致密,磁相互作用增强.这会诱导PS球壳层发生微小的形变,为金属中自由电子提供碰撞的机会.当自由电子与光电场的频率一致时,就会产生较多的SERS热点.而当Fe溅射厚度继续增加时,多层膜的粗糙度就会减小,SERS信号也会随之降低.为了计算半核壳PS@Ag/Fe/Ag活性基底 SERS增强因子,本文采取广泛接受的计算方法,通常情况下平均增强因子定义为:EFave=(ISERS/INR)(NNR/NSERS),ISERS和INR分别为吸附在基底表面PATP分子的SERS特征峰的强度和纯PATP分子的拉曼特征峰的强度.NSERS和NNR分别为基底表面光斑范围内的PATP分子数和固态纯PATP在激光光斑范围内的分子数.PATP单分子层吸附在样品基底上数量可以用NSERS=ARμ计算,其中A为激光光斑照射面积(0.078 5 μm2),R 为基底粗糙因子,μ为基底单位面积内的PATP分子数.粗糙因子R可以用公式R=(SSERS/A)计算,SSERS为激光照射下的SERS活性位点的总面积.固态纯PATP分子数计算公式为NNR=AHρ,ρ为纯液态PATP的分子密度(1.189g/cm3),H为激光照射的有效深度(19 μm),A为激光光斑的照射面积(0.0785 μm2),因此平均增强因子也可以写成:EFave=(Hρ/Rμ)(ISERS/INR).我们对半核壳PS@Ag/Fe/Ag活性基底上的PATP分子的1 430 cm-1峰位进行增强因子计算,得到其增强因子为5×107.本文以自组装的单层PS阵列为模板,设计了一种半核壳PS@Ag/Fe/Ag结构的材料作为SERS活性基底.对不同厚度磁性层测量的结果表明,所有样品均表现出明显的弱磁性,溅射8 nm的Fe层的磁化强度最大.对半核壳PS@Ag/Fe/Ag基底表面进行SERS活性研究结果显示,该阵列具有较强的SERS信号,其增强因子可达到107.磁性层的引入对阵列的SERS效应具有调控作用.鉴于该种半核壳PS@Ag/Fe/Ag结构不仅具有较高的增强因子,还具备磁性这一特性,该种复合材料将会在磁性单元器件制备方面具有潜在的应用价值.【相关文献】[1]FLEISCHM M,HENDRA P J,MCQUILLA A J.Raman spectra of pyridine adsorbed at a silver electrode[J].Chem Phys Lett,1974,26(2):163-166.[2]ZHANG J G,GAO Y,ALVAREZ-PUEBLA R A,et al.Synthesis and SERS properties of nanocrystalline gold octahedra generated from thermal decomposition of HAuCl4 in block copolymers[J].Adv Mater,2006,18(24):3233-3237.[3]ORENDORFF C J,GOLE A,SAU T K,et al.Surface-enhanced raman spectroscopy of self-assembled monolayers:sandwich architecture and nanoparticle shape dependence[J].AnalChem,2005,77(10):3261-3266.[4]NIE S M,EMERY S R.Probing single molecules and single nanoparticles by surface-enhanced raman scattering[J].Science,1997,275(5303):1102-1106.[5]KNEIPP K,WANG Y,DASARI R R.Approach to single-molecule dectection using rhodamine 6G on colloidal silver[J].Appl Spectrosc,1995,49(6):780-784.[6]ZAVALETA C L,SMITH B R,WALTON I,et al.Multiplexed imaging of surface enhanced Raman scattering nanotags in living mice using noninvasive raman spectroscopy[J].Proc Natl Acad Sci U S A,2009,106(32):13511-13516.[7]WANG Y,WEI H,LI B,et al.SERS opens a new way in aptasensor for protein recognition with high sensitivity and selectivity[J].Chem Commun,2007,(48):5220-5222.[8]BRAUN G,LEE S J,DANTE M,et al.Surface-enhanced raman spectroscopy for DNA detection by nanoparticle assembly onto smooth metal films[J].Am ChemSoc,2007,129(20):6378-6379.[9]LI X L,ZHANG Y Z,SHEN Z X.Highly ordered arrays of particle-in-bowl plasmonic nanostructures for surface-enhanced raman scattering[J].Small,2012,8(16):2548-2554. [10]RAUCY J L,LASKER J M.Cell-based systems to assess nuclear receptor activation and their use in drug development[J].Drug Metab Rev,2013,45(1):101-109.[11]ZHANG W H,SCHMID T,YEO B S,et al.Near-field heating,annealing and signal loss in tip-enhanced raman spectroscopy[J].J Phys Chem C,2008,112(6):2104-2108.[12]YANG Z L,AIZPURUA J,XU H X.Electromagnetic field enhancement in TERS configurations[J].Raman Spectrosc,2009,40(10):1343-1438.[13]陈雷,薛向欣,刘洋,等.二氧化硅-金核壳材料的制备与SERS研究[J].吉林师范大学学报(自然科学版),2015,36(4):29-33.。
热重分析(TGA)-完整版资料
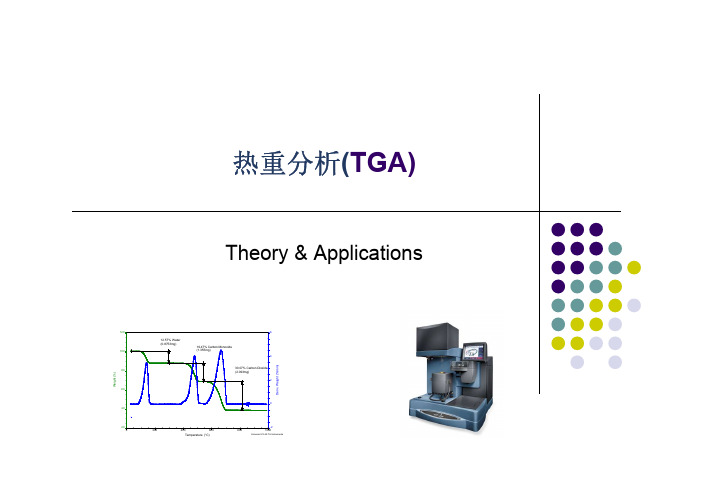
热重分析(TGA)Theory & Applications120612.57% Water (0.8753mg)10019.47% Carbon Monoxide (1.355mg)480Weight (%)30.07% Carbon Dioxide (2.093mg)2600 4020 0 200 400 600 800-2 1000Universal V3.4A TA InstrumentsTemperature (°C)Deriv. Weight (%/min)TGA: The TechniqueThermo gravimetric Analysis (TGA) measures the amount and rate of change in the weight of a material as a function of temperature or time in a controlled atmosphere.•样品在热环境中发生化学变化、分解、成分改变时可能伴随着重量的 变化。
•热重分析就是在不同的热条件(以恒定速度升温或等温条件下延长时 间)下对样品的质量变化加以测量的动态技术。
热重分析的结果用热重曲线或微分热重曲线表示。
2What TGA Can Tell You热稳定性 • Thermal Stability of Materials • Oxidative Stability of Materials 氧化稳定性 • Composition of Multi-component Systems 组成 产品寿命 • Estimated Lifetime of a Product 分解动力学 • Decomposition Kinetics of Materials • The Effect of Reactive or Corrosive Atmospheres 反应或腐蚀气氛对材料的影响 on Materials • Moisture and Volatiles Content of Materials材料水分和挥发份含量3Mechanisms of Weight Change in TGAWeight Loss:Decomposition: The breaking apart of chemical bonds. Evaporation: The loss of volatiles with elevated temperature. Reduction: Interaction of sample to a reducing atmosphere (hydrogen, ammonia, etc). Desorption.Weight Gain:Oxidation: Interaction of the sample with an oxidizing atmosphere. Absorption. All of these are kinetic processes (i.e. there is a rate at which they occur).4TG曲线 积分型曲线DTG曲线 微分型曲线5初始热分解 温度(B、G)最大失重 速率温度反应终了温 度(C、H)6影响热重测定的因素: Purge Gas• TGA: Always purge through balance housing with dry inert gas • TGA: Only introduce reactive/corrosive gases through samplearea/furnace housing • Nitrogen most common. • Helium often provides best baseline but will make furnace work hard at high temperature. • Air can sometimes improve resolution because of differences in the oxidative stability (versus thermal stability) of components. 气氛不同反应机理的不同。
西南科技大学研究了海藻酸铜对高氯酸铵安全性能和热分解性能的增强作用
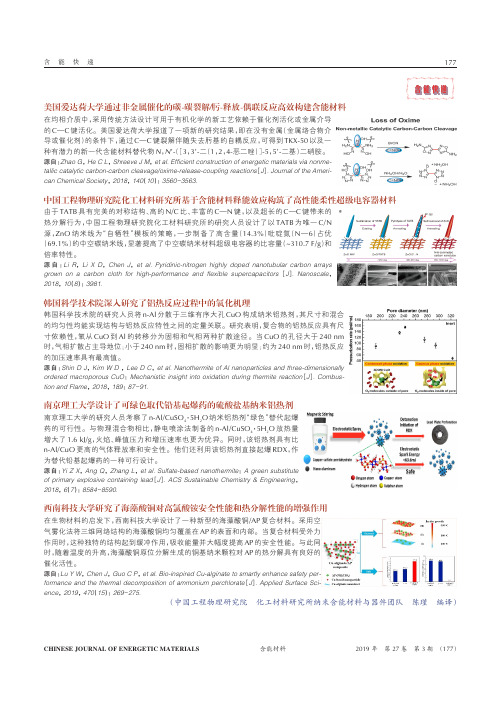
CHINESE JOURNAL OF ENERGETIC MATERIALS 含能材料2019年第27卷第3期(177)含能快递含能快递含能快递美国爱达荷大学通过非金属催化的碳⁃碳裂解/肟⁃释放⁃偶联反应高效构建含能材料在均相介质中,采用传统方法设计可用于有机化学的新工艺依赖于催化剂活化或金属介导的C ─C 键活化。
美国爱达荷大学报道了一项新的研究结果,即在没有金属(金属络合物介导或催化剂)的条件下,通过C ─C 键裂解伴随失去肟基的自耦反应,可得到TKX⁃50以及一种有潜力的新一代含能材料替代物N ,N '⁃([3,3'⁃二(1,2,4⁃恶二唑)]⁃5,5'⁃二基)二硝胺。
源自:Zhao G ,He C L ,Shreeve J M ,et al.Efficient construction of energetic materials via nonme⁃tallic catalytic carbon⁃carbon cleavage/oxime⁃release⁃coupling reactions [J ].Journal of the Ameri⁃can Chemical Society ,2018,140(10):3560-3563.中国工程物理研究院化工材料研究所基于含能材料释能效应构筑了高性能柔性超级电容器材料由于TATB 具有完美的对称结构、高的N/C 比、丰富的C ─N 键,以及超长的C ─C 键带来的热分解行为,中国工程物理研究院化工材料研究所的研究人员设计了以TATB 为唯一C/N源,ZnO 纳米线为“自牺牲”模板的策略,一步制备了高含量(14.3%)吡啶氮(N ─6)占优(69.1%)的中空碳纳米线,显著提高了中空碳纳米材料超级电容器的比容量(~310.7F/g )和倍率特性。
源自:Li R ,Li X D ,Chen J ,et al.Pyridinic⁃nitrogen highly doped nanotubular carbon arraysgrown on a carbon cloth for high⁃performance and flexible supercapacitors [J ].Nanoscale ,2018,10(8):3981.韩国科学技术院深入研究了铝热反应过程中的氧化机理韩国科学技术院的研究人员将n⁃Al 分散于三维有序大孔CuO 构成纳米铝热剂,其尺寸和混合的均匀性均能实现结构与铝热反应特性之间的定量关联。
甲基三苯乙炔基硅烷的制备及固化性能
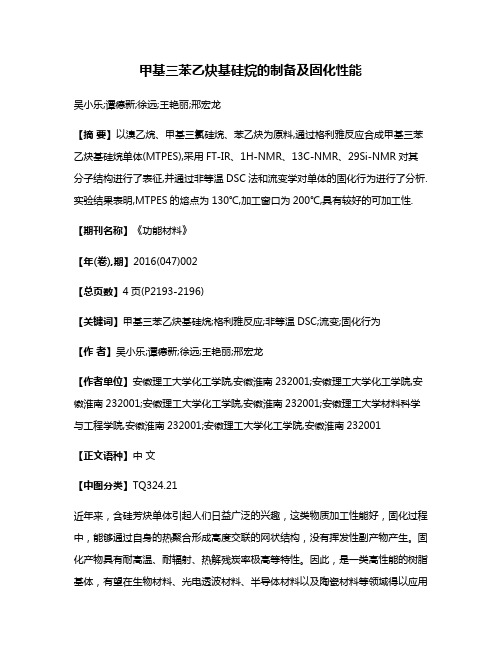
甲基三苯乙炔基硅烷的制备及固化性能吴小乐;谭德新;徐远;王艳丽;邢宏龙【摘要】以溴乙烷、甲基三氯硅烷、苯乙炔为原料,通过格利雅反应合成甲基三苯乙炔基硅烷单体(MTPES),采用FT-IR、1H-NMR、13C-NMR、29Si-NMR对其分子结构进行了表征,并通过非等温DSC法和流变学对单体的固化行为进行了分析.实验结果表明,MTPES的熔点为130℃,加工窗口为200℃,具有较好的可加工性.【期刊名称】《功能材料》【年(卷),期】2016(047)002【总页数】4页(P2193-2196)【关键词】甲基三苯乙炔基硅烷;格利雅反应;非等温DSC;流变;固化行为【作者】吴小乐;谭德新;徐远;王艳丽;邢宏龙【作者单位】安徽理工大学化工学院,安徽淮南232001;安徽理工大学化工学院,安徽淮南232001;安徽理工大学化工学院,安徽淮南232001;安徽理工大学材料科学与工程学院,安徽淮南232001;安徽理工大学化工学院,安徽淮南232001【正文语种】中文【中图分类】TQ324.21近年来,含硅芳炔单体引起人们日益广泛的兴趣,这类物质加工性能好,固化过程中,能够通过自身的热聚合形成高度交联的网状结构,没有挥发性副产物产生。
固化产物具有耐高温、耐辐射、热解残炭率极高等特性。
因此,是一类高性能的树脂基体,有望在生物材料、光电透波材料、半导体材料以及陶瓷材料等领域得以应用[1-6]。
关于含硅芳炔单体的合成已有诸多报道。
Wrackmeyer[7]将其分为两类:一为氯硅烷与格氏试剂(或者炔基锂试剂)的传统反应方法;二为多步复杂的其它反应方法。
后者,较为常用的是偶合脱氢反应,如以氢硅烷、炔基化合物为原料,金属化合物(如H2PtCl6/LiI,IrH2(SiEt3)(COD)(AsPh3)、[IrH(H2O](bp)L2)]SbF6、RhClL3 (L=PPh, bp=7, 8-benzoquinolinato))为催化剂的偶合脱氢[8]。
电石渣与石灰石热分解特性比较及电石渣热分解动力学
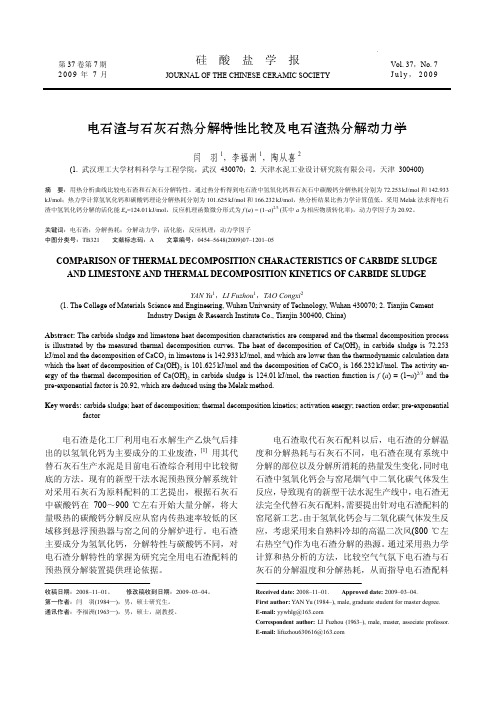
马贺等:水热合成β-MnO2纳米棒的电磁特性· 1201 ·第36卷第7期电石渣与石灰石热分解特性比较及电石渣热分解动力学闫羽1,李福洲1,陶从喜2(1. 武汉理工大学材料科学与工程学院,武汉 430070;2. 天津水泥工业设计研究院有限公司,天津 300400)摘要:用热分析曲线比较电石渣和石灰石分解特性。
通过热分析得到电石渣中氢氧化钙和石灰石中碳酸钙分解热耗分别为72.253kJ/mol和142.933 kJ/mol;热力学计算氢氧化钙和碳酸钙理论分解热耗分别为101.625kJ/mol和166.232kJ/mol,热分析结果比热力学计算值低。
采用Melak法求得电石渣中氢氧化钙分解的活化能E a=124.01kJ/mol,反应机理函数微分形式为f (a) = (1–a)2/3 (其中a为相应物质转化率),动力学因子为20.92。
关键词:电石渣;分解热耗;分解动力学;活化能;反应机理;动力学因子中图分类号:TB321 文献标志码:A 文章编号:0454–5648(2009)07–1201–05COMPARISON OF THERMAL DECOMPOSITION CHARACTERISTICS OF CARBIDE SLUDGE AND LIMESTONE AND THERMAL DECOMPOSITION KINETICS OF CARBIDE SLUDGEYAN Yu1,LI Fuzhou1,TAO Congxi2(1. The College of Materials Science and Engineering, Wuhan University of Technology, Wuhan 430070; 2. Tianjin CementIndustry Design & Research Institute Co., Tianjin 300400, China)Abstract: The carbide sludge and limestone heat decomposition characteristics are compared and the thermal decomposition process is illustrated by the measured thermal decomposition curves. The heat of decomposition of Ca(OH)2 in carbide sludge is 72.253 kJ/mol and the decomposition of CaCO3 in limestone is 142.933kJ/mol, and which are lower than the thermodynamic calculation data which the heat of decomposition of Ca(OH)2 is 101.625kJ/mol and the decomposition of CaCO3 is 166.232kJ/mol. The activity en-ergy of the thermal decomposition of Ca(OH)2 in carbide sludge is 124.01kJ/mol, the reaction function is f (a) = (1–a)2/3 and the pre-exponential factor is 20.92, which are deduced using the Melak method.Key words: carbide sludge; heat of decomposition; thermal decomposition kinetics; activation energy; reaction order; pre-exponential factor电石渣是化工厂利用电石水解生产乙炔气后排出的以氢氧化钙为主要成分的工业废渣,[1]用其代替石灰石生产水泥是目前电石渣综合利用中比较彻底的方法。
MSDS英文模板(1)
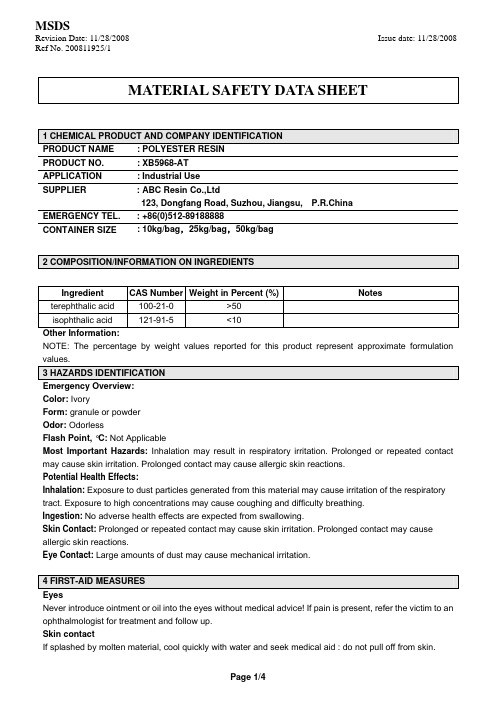
MATERIAL SAFETY DATA SHEET1CHEMICAL PRODUCT AND COMPANY IDENTIFICATIONPRODUCT NAME : POLYESTER RESINPRODUCT NO. : XB5968-ATUseAPPLICATION :IndustrialSUPPLIER : ABC Resin Co.,Ltd123, Dongfang Road, Suzhou, Jiangsu, P.R.ChinaEMERGENCY TEL. : +86(0)512-89188888CONTAINER SIZE : 10kg/bag,25kg/bag,50kg/bag2 COMPOSITION/INFORMATION ON INGREDIENTSIngredient CAS Number Weight in Percent (%)Notesterephthalic acid 100-21-0 >50isophthalic acid 121-91-5 <10Other Information:NOTE: The percentage by weight values reported for this product represent approximate formulation values.3 HAZARDS IDENTIFICATIONEmergency Overview:Color: IvoryForm: granule or powderOdor: OdorlessFlash Point, °C: Not ApplicableMost Important Hazards: Inhalation may result in respiratory irritation. Prolonged or repeated contact may cause skin irritation. Prolonged contact may cause allergic skin reactions.Potential Health Effects:Inhalation: Exposure to dust particles generated from this material may cause irritation of the respiratory tract. Exposure to high concentrations may cause coughing and difficulty breathing.Ingestion: No adverse health effects are expected from swallowing.Skin Contact: Prolonged or repeated contact may cause skin irritation. Prolonged contact may cause allergic skin reactions.Eye Contact: Large amounts of dust may cause mechanical irritation.4 FIRST-AID MEASURESEyesNever introduce ointment or oil into the eyes without medical advice! If pain is present, refer the victim to an ophthalmologist for treatment and follow up.Skin contactIf splashed by molten material, cool quickly with water and seek medical aid : do not pull off from skin.5 FIRE-FIGHTING MEASURESGeneral informationAs in any fire, wear a self-contained breathing apparatus in pressure-demand and full protective gear. During a fire, irritating and highly toxic gases may be generated by thermal decomposition or combustion Suitable extinguishing agentsIn case of fire, use sand, carbon dioxide or powdered extinguishing agent.Special hazards caused by the material, its products of combustion or resulting gases.Carbon monoxide (CO)Carbondioxide(CO2)Protective equipmentMount respiratory protective device6 ACCIDENTAL RELEASE MEASURESPerson-related safety precautionsUse proper personal protective equipment as Exposure controls and personal protection.Measures for environmental protectionDo not allow to enter sewers/ surface or ground water.Small SpillUse appropriate tools to put the spilled solid in a convenient waste disposal container. Finish cleaning by spreading water on the contaminated surface and dispose of according to local and regional authority requirements.Large SpillUse a shovel to put the material into a convenient waste disposal container. Be careful that the product is not present at a concentration level above TLV.7 HANDLING AND STORAGEHandlingHandle in accordance with good industrial hygiene and safety practices. These practices include avoiding unnecessary exposure and removal of material from eyes, skin and clothing.Engineering measuresProvide natural or mechanical ventilation to minimize exposure. If practical, use local mechanical exhaust ventilation at sources of air contamination such as processing equipment.StorageStable under normal conditions of handling and storage.8 EXPOSURE CONTROLS/PERSONAL PROTECTIONOccupational exposure limitNational authorities have established limits for particulates not otherwise classified that are the least stringent exposure limits applicable to dusts.ACGIH TLV: 10mg/m3 (total dust) 8-hr TWAACGIH TLV: 3mg/m3 (respirable) 8-hr TWAPersonal Protective EquipmentSafety glasses with side shields. Wear suitable gloves.Respiratory ProtectionUse approved respiratory protection if exposure limits are exceeded, or overexposure is likely. If respiratory protection is used, follow all requirements for respiratory programs set forth in government regulations. VentilationGeneral ventilation. Local exhaust.9 PHYSICAL AND CHEMICAL PROPERTIESGeneral InformationForm: SoildColour: White/IvoryOdour: OdorlessChange in conditionMelting point/Melting range: undeterminedBoiling point/Boiling range: undeterminedFlash point: Not applicableSelf igniting: Product is not self igniting.Danger of explosion: Product does not present an explosion hazard.10 STABILITY AND REACTIVITYConditions to avoidNone knownMaterials to avoidNone knownHazardous reactionsHazardous polymerization does not occur.Hazardous decomposition productsConditions: Decomposition occurs above temperature listed below :Decomposition temperature: > 300℃Decomposition products: carbon monoxide (CO), carbon dioxide(CO2)11 TOXICOLOGICAL INFORMATIONLow toxicityPrimary irritant effecton the skin: Prolonged and/or repeated skin contact may defat skin and possibly cause dermatitis on the eye: Low, reversible irritation.SensitizationNone known.Additional toxicological informationWhen used and handled according to specifications, the product does not have any harmful effects according to our experience and the information provided to us.12 ECOLOGICAL INFORMATIONEcotoxicity: Not available.BOD5 and COD: Not availableProducts of BiodegradationPossibly hazardous short term degradation products are not likely. However, long term degradation products may Arised.13 DISPOSAL CONSIDERATIONSIncineration, RecycleAll local and national regulations should be followed.14 TRANSPORT INFORATIONROAD/RAILOther Not regulated for transport.SEANot regulated for transport.AIRNot regulated for transport.15 REGULATORY INFORMATIONEC labelEU Dangerous Substances Directive 67/548/EC.16 OTHER INFORMATIONThis product may contain other copolymers, colour additives, heat stabilizers, flame retardants and/or other performance additives. Under normal use conditions, these additives are contained within the polymer matrix and occupational exposures are expected to be minimal.Data sheets prepared or revised have been completed in accordance with the European Communities Directive 2001/58.Although the information and recommendations set forth herein (hereinafter "Information") are presented in good faith and believed to be correct as of the date hereof, The company makes no representations as to the completeness or accuracy thereof. Information is supplied upon the condition that the persons receiving same will make their own determination as to its suitability for their purposes prior to use. In no event will The company be responsible for damages of any nature whatsoever resulting from the use of or reliance upon Information. NO REPRESENTATIONS OR WARRANTIES, EITHER EXPRESS OR IMPLIED, OF MERCHANTABILITY, FITNESS FOR A PARTICULAR PURPOSE OR OF ANY OTHER NATURE ARE MADE HEREUNDER WITH RESPECT TO INFORMATION OR THE PRODUCT TO WHICH INFORMATION REFERS.。
4-Introduction

2
Part 5
Writing Methods for Introduction
3
Part 5 Introduction
Introduction
❖ The introduction is the first portion of the text. Though it is often preceded by an abstract, the introduction should be a complete and selfsufficient unit with one or more paragraphs.
aromatic 芳香族; aliphatic 脂肪族
12
Part 5 Introduction
一.简介研究背景(background)﹑ 研究意义
❖ Since the early 1960s, …has attracted much attention due to …
❖ In recent years, more and more attention has been paid to …
14
Part 5 Introduction
二. 论述目前该领域旳研究现状﹑存在问题﹑待处理旳问题
❖ Although the cationic ring-opening polymerization of SOCs has been investigated extensively, only a low molecular weight (MW) polymer was obtained and the MW cannot be controlled.
❖ The free radical polymerizations of cyclic ketene (烯酮)acetals (乙缩醛,乙缩醛二乙醇)have recently evoked(唤起) a lot of interest.
煤化学 英语课件chapter_9_thermal_pyrolysis_of_coal
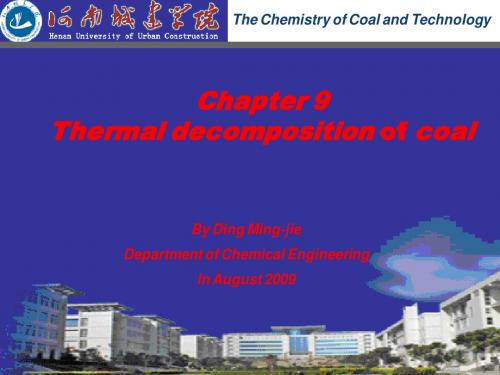
2009/08
Temperature stages
Below 100℃---- the formation of low molecular weight species: water ℃ absorbed methane and carbon dioxide 100-200℃--- more than 50% of the carboxylic acid functions can lose ℃ carbon dioxide 200-370℃--- coals lose a variety of lower molecular weight organic ℃ species (may aliphatic compounds, and some of the aromatic species ) which are believed to arise from moieties that are “loosely bound” to the more thermally stable part of the coal structure. proper 350℃---- cracking temperature ℃ >370℃; >700℃--- methane (indicative of the thermal destruction of ℃ ℃ the coal structure), polycyclic aromatics, phenols, and nitrogen compounds are produced. For bituminous coals, the decomposition increases markedly above 400℃ and reaches a maximum in the range 700-900℃ ℃ ℃
【学习】第2章-聚合物燃烧与成炭

❖ Substituent Reactions
Elimination, Cyclization
Structural defects Reactive impurities “Special” structures (e.g. chain end, etc.) “Weak links”
.
北京理工大学
2.1 聚合物热分解过程及机理
.
北京理工大学
2.1 聚合物热分解过程及机理
Thermal decomposition: a process of extensive chemical species change
caused by heat Thermal degradation:
a process whereby the action of heat or elevated temperature on a material, product, or assembly causes a loss of physical, mechanical, or electrical properties
了解聚合物燃烧行为及产物;阻燃剂的选择及配方设计; 指导新型阻燃材料的研究…..
五氟乙烷灭火剂高温热解机理研究_冒爱琴

第48卷第1期Vol.48No.1山东大学学报(理学版)Journal of Shandong University (Natural Science )2013年1月Jan.2013收稿日期:2012-10-16;网络出版时间:2012-12-1818ʒ04网络出版地址:http ://www .cnki.net /kcms /detail /37.1389.N.20121228.1804.006.html基金项目:国家科技支撑项目(2008BAK40B02)作者简介:冒爱琴(1978-),女,讲师,博士研究生,研究方向为有机氟化学合成及催化剂设计.Email :maoaiqinmaq@163.com *通讯作者:潘仁明(1963-),男,博士,研究员,博士生导师,研究方向为新型灭火剂和含能材料.Email :panrenming@163.com文章编号:1671-9352(2013)01-0051-05DOI :10.6040/j.issn.1671-9352.0.2012.528五氟乙烷灭火剂高温热解机理研究冒爱琴1,2,杨明君2,3,俞海云2,张品1,潘仁明1*(1.南京理工大学化工学院,江苏南京210094;2.安徽工业大学材料科学与工程学院,安徽马鞍山243002;3.哥本哈根大学化学系纳米科学中心,丹麦哥本哈根DK-2100)摘要:根据均裂的化学键类型提出了五氟乙烷(C 2HF 5)的9条初级裂解反应路径,采用DFT /B2LYP /6-31G *、DFT /B2LYP /6-311G **、MP2/6-31G *和MP2/6-311G **方法计算了C 2F 5H 各裂解反应的焓变、中间态分子模型以及反应中生成的卡宾的能态。
结果表明C 2HF 5裂解最易发生H 转移反应生成C 2F 4;伴随H 转移生成CF 3CF :卡宾,F 转移生成CHF :,C —C 键断裂生成CF 2H ·和CF 3·,自由基或卡宾之间相互结合生成微量的C 2F 6、C 4F 8和C 4F 10等气体产物。
- 1、下载文档前请自行甄别文档内容的完整性,平台不提供额外的编辑、内容补充、找答案等附加服务。
- 2、"仅部分预览"的文档,不可在线预览部分如存在完整性等问题,可反馈申请退款(可完整预览的文档不适用该条件!)。
- 3、如文档侵犯您的权益,请联系客服反馈,我们会尽快为您处理(人工客服工作时间:9:00-18:30)。
[
RA(n-1)(' "(i __ C~,)n I ~" 13E2 ,
1
TB(E-2RT)
e (~n
~Z(E - 2RT.) )]1/(,, 1)
e {~:/eT>')
(8)
Assuming that we could obtain the activation energies of copolymers with the additivity rule and activation energies of PMMA, PEMA and PBMA, those could be as the follows,
INTRODUCTION
Continuous process offers the advantages of improved polymer properties and economies of scale but the design of continuous systems requires more understanding than that required in the design of batch system [-1-6]. In the meantime, conversions are generally low in the continuous process and the kinetics of a polymerization reaction are not simple [3]. Our previous work was reported on the continuous copolymerization of methyl methacrylate with alkyl methacwlates [7]. It was also reported that the unzipping property of methyl methacwlate can be prevented by incorporating second monomer through a copolymerization. The previous study revealed that the copolymerization of MMA with EMA or BMA followed the second-order kinetics in a CSTR, regardless of the compositions of the copolymers. The present paper deals with the thermal degradation behavior of the MMA/EMA or MMA/BMA copolymers, which were obtained from our previous CSTR experiments. We have investigated the following axioms: the copolymerization process of MMA and alkyl methacwlate may follow a reverse order of the unzipping process during the thermal degradation, and lhen the copolymerization kinetics may be almost the same as *To whom all correspondences should be addressed. 74
Thermal Decompn. of Copolym. of MMA and Alkyl MA Obtained from a CSTR
75
t - time A=pre-exponential factor of rate constant E=activation energy of rate constant R = gas constant T = absolute temperature Taking logarithms of Eq. (1) gives ln(~-t )=lnA+ln(1-c)"-~E'~lR T (2)
n
1
c)" 1 (1_c),_~.(1_<,)._~ = _ , Xo- RT, me x
fx X
[P(X)-P(Xo)] (5)
where, X = e x P(X) = ~ e Xo
-
where, n is a kinetic order of the thermal degradation. Taking parameters of the heating rate (13=dT/dt) in the given conversion, we obtained activation energy, E from the graph slopes which is related to ln(dc/dt) and 1/T of Eq. (2). The kinetic order (n) and pre-exponential factor (A) were calculated from the slopes and intercepts of the
THEORY
Kinetics of thermal degradation of polymers have been evaluated from thermogravimetric analysis (TGA) at linear rates of temperature rise in a number of studies [8-13]. In the present paper, kinetic calculations are based on the intercomparison of experiments which were carried out at several different rates of temperature rise according to the Friedman's method [10]. The general kinetic equation of thermal degradation of polymers is dc dt - A" ( 1 - cY" expl where, c degree of conversion (1)
Taking integration of Eq. (4) in the given boundary condition (from C=Co, T=To to c=co, T = T ) , which gives
1 ( 1 - - Cv) n 1__ ( 1 -
Let the term, f ( 1 - c ) be (1-c)" of Eq. (2) and multiply pre-exponential factor and taking logarithms to both sides, which gives ln[A. f(1 - c)] = In A + n In(1 - c) (3)
Korean J. of Chem. Eng., 11(2), 74-80(1994)
THERMAL DECOMPOSITION OF COPOLYMERS OF METHYL METHACRYLATE AND ALKYL METHACRYLATES OBTAINED FROM A CSTR
Bong Sub Shin and Soo Duk Seul*
Dept. of Chem. Eng., Dong-A Univ., Pusan 604-714, Korea (Received 15 February 1993 9 accepted 24 November 1993)
A b s t r a c t - T h e r m a l decomposition of the copolymers of methyl methacrylate (MMA) with ethyl methacrylate (EMA) or n-butyl rnethacrylate (BMA) were investigated. The eopolymers were obtained in a continuous stirred tank reactor (CSTR) using toluene and benzoyl peroxide, as solvent and initiator, respectively, at 80C. The w~lume was 1.2 litlers and residence time was 3 hours. The thermal decomposition followed the second order kinetics for both MMA/EMA and MMA/BMA copolymers, which were almost in accordance with the order of copolymerization in a CSTR. The activation energies of thermal decomposition were in the ranges of 32-37 kcal/mol and 27-37 kcal/mole for MMA/EMA and MMA/BMA copolymers, respectively and a good additivity rule was observed against each composition for both copolymers. The thermogravimetric trace curve agreed well with the theoretical calculation.