g-C3N4的制备及表征
g-C_(3)N_(4)光催化剂的改性及制备
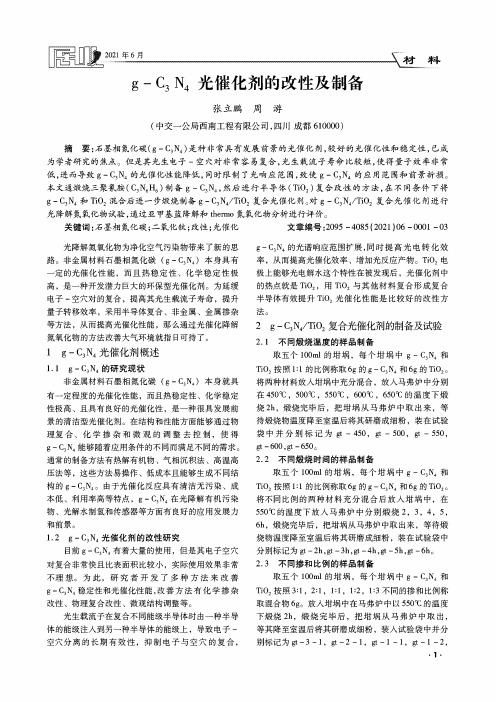
Im———二.—
g - C3 n4光催化剂的改性及制备
张立鹏周游
(中交一公局西南工程有限公司,四川成都610000)
摘 要:石墨相氮化碳(g-C3N4)是种非常具有发展前景的光催化剂,较好的光催化性和稳定性,已成 为学者研究的焦点。但是其光生电子-空穴对非常容易复合,光生载流子寿命比较短,使得量子效率非常
解率,通过试验结果可以得出g - C3N4/TiO2复合光 催化剂对氮氧化物的降解效率较g - C3N4和TiO2的 单体提高了大约7%。
参考文献:
[1] 冯西平,张宏,杭祖圣.g-G3N4及改性g-C3N4的 光催化研究进展[J].功能材料与器件学报,2012, 18 (3) : 214-222.
2.1不同锻烧温度的样品制备 取五个100ml的堆竭,每个堆竭中g - C3N4和
TiO2按照1 : 1的比例称取6g的g-C3N4和6g的TiO2。 将两种材料放入堆竭中充分混合,放入马弗炉中分别 在450兀,500兀,550兀,600兀,650兀的温度下锻 烧2h,锻烧完毕后,把堆竭从马弗炉中取出来,等 待锻烧物温度降至室温后将其研磨成细粉,装在试验 袋中并分别标记为gt - 450, gt - 500, gt - 550, gt - 600, gt -650o 2.2不同锻烧时间的样品制备
5 结语
综上所述,观我国现阶段的建筑业发展情况来 看,无论是建筑高度还是建筑规模都在与日俱增,而 随着一栋栋“摩天大楼”的拔地而起,对其设计也 提出了更高的要求。在超高层建筑设计中,伸臂加强 层结构设计是一项重难点问题,特别是超高层建筑伸 臂加强层结构设置位置的选择十分关键,必须要保证 伸臂加强层结构设计满足建筑的使用功能需求且符合 相关结构控制目标,同时还要确保伸臂桁架构件尺寸 合理,可承受适当作用力,方便施工作业。
热还原法制备g-C3N4/高岭石复合材料及其光/过硫酸盐协同催化性能
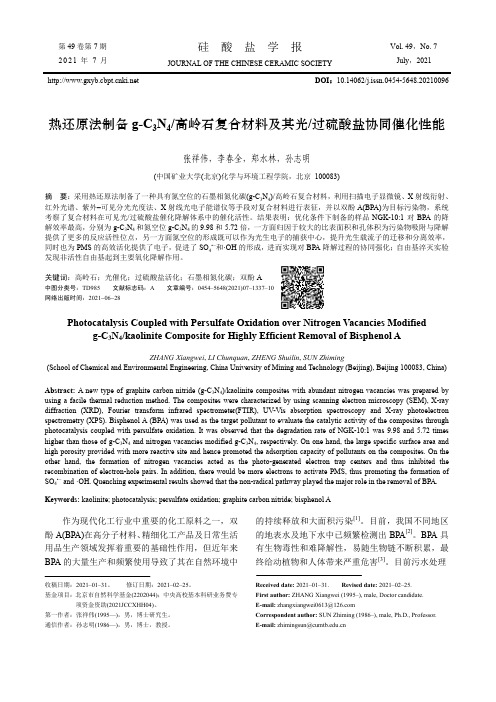
第49卷第7期2021年7月硅酸盐学报Vol. 49,No. 7July,2021 JOURNAL OF THE CHINESE CERAMIC SOCIETY DOI:10.14062/j.issn.0454-5648.20210096 热还原法制备g-C3N4/高岭石复合材料及其光/过硫酸盐协同催化性能张祥伟,李春全,郑水林,孙志明(中国矿业大学(北京)化学与环境工程学院,北京 100083)摘要:采用热还原法制备了一种具有氮空位的石墨相氮化碳(g-C3N4)/高岭石复合材料,利用扫描电子显微镜、X射线衍射、红外光谱、紫外-可见分光光度法、X射线光电子能谱仪等手段对复合材料进行表征,并以双酚A(BPA)为目标污染物,系统考察了复合材料在可见光/过硫酸盐催化降解体系中的催化活性。
结果表明:优化条件下制备的样品NGK-10:1对BPA的降解效率最高,分别为g-C3N4和氮空位g-C3N4的9.98和5.72倍,一方面归因于较大的比表面积和孔体积为污染物吸附与降解提供了更多的反应活性位点,另一方面氮空位的形成既可以作为光生电子的捕获中心,提升光生载流子的迁移和分离效率,同时也为PMS的高效活化提供了电子,促进了SO4•−和·OH的形成,进而实现对BPA降解过程的协同强化;自由基淬灭实验发现非活性自由基起到主要氧化降解作用。
关键词:高岭石;光催化;过硫酸盐活化;石墨相氮化碳;双酚A 中图分类号:TD985 文献标志码:A 文章编号:0454–5648(2021)07–1337–10网络出版时间:2021-06-28Photocatalysis Coupled with Persulfate Oxidation over Nitrogen Vacancies Modified g-C3N4/kaolinite Composite for Highly Efficient Removal of Bisphenol AZHANG Xiangwei, LI Chunquan, ZHENG Shuilin, SUN Zhiming(School of Chemical and Environmental Engineering, China University of Mining and Technology (Beijing), Beijing 100083, China) Abstract: A new type of graphite carbon nitride (g-C3N4)/kaolinite composites with abundant nitrogen vacancies was prepared by using a facile thermal reduction method. The composites were characterized by using scanning electron microscopy (SEM), X-ray diffraction (XRD), Fourier transform infrared spectrometer(FTIR), UV-Vis absorption spectroscopy and X-ray photoelectron spectrometry (XPS). Bisphenol A (BPA) was used as the target pollutant to evaluate the catalytic activity of the composites through photocatalysis coupled with persulfate oxidation. It was observed that the degradation rate of NGK-10:1 was 9.98 and 5.72 times higher than those of g-C3N4 and nitrogen vacancies modified g-C3N4, respectively. On one hand, the large specific surface area and high porosity provided with more reactive site and hence promoted the adsorption capacity of pollutants on the composites. On the other hand, the formation of nitrogen vacancies acted as the photo-generated electron trap centers and thus inhibited the recombination of electron-hole pairs. In addition, there would be more electrons to activate PMS, thus promoting the formation of SO4•− and ·OH. Quenching experimental results showed that the non-radical pathway played the major role in the removal of BPA.Keywords: kaolinite; photocatalysis; persulfate oxidation; graphite carbon nitride; bisphenol A作为现代化工行业中重要的化工原料之一,双酚A(BPA)在高分子材料、精细化工产品及日常生活用品生产领域发挥着重要的基础性作用,但近年来BPA的大量生产和频繁使用导致了其在自然环境中的持续释放和大面积污染[1]。
一种多孔g-c3n4光催化剂的制备方法与流程

一种多孔g-c3n4光催化剂的制备方法与流程多孔g-C3N4光催化剂是一种具有较大比表面积和孔隙结构的材料,在可见光催化反应中具有较高的催化活性。
下面将介绍一种常用的多孔g-C3N4光催化剂的制备方法与流程。
首先,准备所需的原料和试剂。
制备多孔g-C3N4光催化剂的主要原料是氯化铜(CuCl2)、尿素(CO(NH2)2)和溶剂。
在制备过程中还需要使用一些辅助试剂,例如硝酸铝(Al(NO3)3)和过氧化氢(H2O2)。
第一步,制备前驱体。
将CuCl2溶解在足够的溶剂中,通常可以选择水或有机溶剂。
随后,将尿素加入到溶液中,并进行充分搅拌,直到溶液成为均匀的悬浮液。
第二步,形成前驱体膜。
将悬浮液倒入玻璃或陶瓷容器中,然后将其放置在烘箱中进行干燥。
通过调节干燥的温度和时间,可以控制前驱体的结晶度和形貌。
第三步,热处理。
将干燥后的前驱体膜放入炉中进行热处理,一般在氮气气氛下进行。
热处理的温度和时间是控制多孔g-C3N4形貌和结构的关键因素。
通常,较高的温度和长时间的处理会产生具有较大比表面积和孔隙结构的多孔g-C3N4。
第四步,浸泡法。
将热处理得到的多孔g-C3N4样品浸入溶液中,通常使用硝酸铝、过氧化氢等试剂。
浸泡时间一般为几小时到几天不等,可以根据需要调整。
第五步,洗涤和干燥。
将浸泡后的样品取出,用大量的溶液进行洗涤,去除残留的试剂和杂质。
然后,将样品放置在烘箱中进行干燥,得到最终的多孔g-C3N4光催化剂。
最后,对制备得到的多孔g-C3N4进行表征和应用。
可以使用扫描电子显微镜(SEM)、透射电子显微镜(TEM)、X射线衍射(XRD)等技术对样品的形貌、结构和成分进行表征。
同时,也可以将其应用于可见光催化反应中,例如光催化水分解、光催化有机污染物降解等。
需要注意的是,这只是一种传统的多孔g-C3N4光催化剂制备方法与流程,实际制备过程中还可以根据需要进行改进和优化。
不同的制备条件可能会对多孔g-C3N4的形貌和结构产生不同的影响,因此,制备过程中需要进行合理的实验设计和参数调控。
石墨相氮化碳的化学合成及应用

石墨相氮化碳的化学合成及应用一、本文概述石墨相氮化碳(g-C3N4)是一种新兴的二维纳米材料,自其被发现以来,已在科学界引起了广泛的关注。
本文旨在深入探讨石墨相氮化碳的化学合成方法以及其在多个领域的应用。
我们将首先概述石墨相氮化碳的基本性质,然后详细介绍其化学合成的最新进展,最后探讨其在能源转换、环境修复、生物医学等领域的应用前景。
通过对石墨相氮化碳的深入研究,我们期待能够为材料科学的发展提供新的思路和方法,同时也为实际问题的解决提供有效的材料基础。
二、石墨相氮化碳的化学合成石墨相氮化碳(g-C3N4)是一种新兴的二维纳米材料,因其独特的电子结构和物理化学性质,在能源转换、光催化、传感器等领域具有广泛的应用前景。
其合成方法多种多样,主要包括热缩聚法、溶剂热法、气相沉积法以及微波辅助法等。
热缩聚法:热缩聚法是最常见的制备g-C3N4的方法之一。
通常,富含氮的前驱体(如尿素、硫脲、三聚氰胺等)在高温下(如500-600℃)进行热缩聚,生成g-C3N4。
这种方法简单易行,产量大,但得到的g-C3N4往往结晶度不高,存在大量的结构缺陷。
溶剂热法:溶剂热法是一种在溶剂存在下进行热缩聚的方法。
通过选择合适的溶剂和反应条件,可以调控g-C3N4的形貌和结构。
这种方法制备的g-C3N4通常具有较高的结晶度和较大的比表面积。
气相沉积法:气相沉积法是一种在基底上直接生长g-C3N4的方法。
通过控制气相反应的条件,可以在基底上制备出高质量的g-C3N4薄膜。
这种方法制备的g-C3N4具有良好的结晶度和均匀的厚度,适用于制备大面积、高质量的g-C3N4。
微波辅助法:微波辅助法是一种利用微波加热快速合成g-C3N4的方法。
微波加热具有快速、均匀、节能等优点,可以在短时间内完成g-C3N4的合成。
这种方法制备的g-C3N4具有较高的结晶度和良好的分散性。
除了以上几种方法外,还有一些其他的合成方法,如电化学合成法等离子体法等。
g-C3N4增强木塑的制备和表征

Preparation and characterization of wood–plastic composite reinforced by graphitic carbonnitrideBingrong Lei a ,Yaru Zhang a ,Yanjin He a ,Yongfeng Xie a ,Baiping Xu b ,Zhidan Lin d ,Langhuan Huang a ,Shaozao Tan a ,⇑,Meigui Wang b ,⇑,Xiang Cai c ,⇑aDepartment of Chemistry,Jinan University,Guangzhou 510632,ChinabDepartment of Light Chemical Engineering,Guangdong Industry Technical College,Guangzhou 510300,China cDepartment of Light Chemical Engineering,Guangdong Polytechnic,Foshan 528041,China dDepartment of Materials Science and Engineering,Jinan University,Guangzhou 510632,Chinaa r t i c l e i n f o Article history:Received 9June 2014Accepted 16October 2014Available online 24October 2014Keywords:Wood plastic composite Graphitic carbon nitride Mechanical properties Thermal properties Outward colora b s t r a c tThe aim of this study was to evaluate and characterize various properties of experimental composition prepared from graphitic carbon nitride (g-C 3N 4),wood flour (WF)and polypropylene (PP).g-C 3N 4with different concentrations (1wt.%,3wt.%,5wt.%and 10wt.%)were used as reinforcing filler for wood plas-tic compositions (WPCs).Maleic anhydride grafted polypropylene (PP-g-MA)was added as a coupling agent to increase the interaction between the components.Water absorption,morphology,physical,mechanical and thermal properties of the as-prepared composites were evaluated.The results showed that the tensile modulus of the composite was increased by 142.9%with increasing of g-C 3N 4contents to 5wt.%,reaching approximately 498MPa compared to WPC.Moreover,the flexural and tensile strengths reached their maximum values when the concentrations of g-C 3N 4were 1wt.%and 3wt.%,respectively.When maintaining the g-C 3N 4at a low concentration,it was well dispersed in the WPC with thin plate shape.However,when more g-C 3N 4(3–10wt.%)was introduced,the enhancing effect began to diminish because of the agglomeration of g-C 3N 4which caused poor interfacial adhesion.The water absorption results showed a lower value with the addition of 1wt.%g-C 3N 4,and the thermal tests showed that the degradation temperature shifted to higher value clearly after the addition of g-C 3N 4.Besides,with the addition of g-C 3N 4improved the outward color compared to the control sample.Crown Copyright Ó2014Published by Elsevier Ltd.All rights reserved.1.IntroductionWood flour (WF)is gaining more and more acceptance as a kind of filler for polymers due to its low density,easy availability,bio-degradation,high stiffness,renewability and relatively low cost.In addition,the renewable and biodegradable characteristics of wood fibers facilitate their ultimate disposal by composting or incineration.According to the advantages of wood fiber,the production of wood plastic composites (WPCs)and its application in many areas has attracted much attention in the past ten years [1].They were used in interior decoration and construction indus-tries such as decking,railing,fencing,docks,landscaping timbers,and in a number of automobile industries [2].However,when com-bining thermoplastics with wood fibers by conventional methods,the highly hydrophilic characters of the lignocelluloses materials make them incompatible with the thermoplastics which are highly hydrophobic.The incompatibility leads to poorer interfacial adhesion between thermoplastics and wood filler,and worse of the composite properties.In addition,the hydroxyl groups between wood fibers can form hydrogen bonds which can lead to agglomeration the fibers into bundles and unevenly distribution throughout the non-polar polymer matrix during the compound-ing processing [3,4].However,the WF is mainly made of cellulose,hemicelluloses,lignin and pectins,which leads to water absorption of the WPC resulting in debonding fibers and degradation of the fiber–matrix interface.In addition,the high moisture absorption of natural fibers may cause dimensional change of the resulting composite and weakened the interfacial adhesion [5,6].WPC in the extrusion process are mainly affected by the barrel tempera-ture and the die temperature.Due to the high temperate leads to the carbonization of WF,the appearance color of the products is affected.Many studies were devoted to enhancing the physical and mechanical properties of these composite materials,such as the/10.1016/j.matdes.2014.10.0410261-3069/Crown Copyright Ó2014Published by Elsevier Ltd.All rights reserved.⇑Corresponding authors.E-mail addresses:tanshaozao@ (S.Tan),1987103016@ (M.Wang),cecaixiang@ (X.Cai).tensile and flexural strengths [7].Usually there areto accomplish this through the use of different the filler can be treated with coupling agent or terms of the particle size,such as processing WF [8],silane treatment [9],heat treatment [10],or sodium hydroxide [11].On the other hand,using such as nanoclay or carbon nanotubes is one of to reinforce the mechanical properties of the nano-materials have positive results including increased tensile and flexural strength,high low water absorption [12,13].Many researchers the nanoclay as nanofiller to improve physical properties of WPC [12,14].In the recent years,nitride (g-C 3N 4)has been widely used in many catalytic areas,especially for oxygen reduction reaction since Wang et al.have founded its photocatalytic ability to produce hydrogen from a methanol aqueous solution under visible-light irradiation [15].However,to the best of our knowledge,g-C 3N 4has not been used as reinforcement for WPC.In this study,pure melamine was used to prepared the g-C 3N 4by directly heating [16].The main objective of this work was to study the effect of g-C 3N 4as a reinforcing agent on the physico-mechanical properties of WPC.2.Experimental details 2.1.MaterialsMelamine was purchased from Tianjin Municipality kemi’ou Chemical Reagent Co.,Ltd.;PP (T30S)was purchased from China Petroleum Chemical Co.,Ltd.(Maoming,China);the satiric acid (lubricant)and coupling agent (PP-g-MA,grafting degree was 0.8%)were supplied by Ma Ji Sen composite materials Co.,Ltd.;wood flour (WF,<150l m)was supplied by Wei Hua spice Co.,Ltd.(Guangdong,China).2.2.Preparation of g-C 3N 4The g-C 3N 4was prepared by simple calcination of melamine powder under air atmosphere.First,an certain amount of the mel-amine power were put into a semi-closed alumina crucible with a cover.Second,the crucible was heated to 550°C for 2h,at a heat-ing rate of 2°C min À1.After cooling to room temperature,the obtained yellow sample was grinded into powder and dried at 85°C.2.3.Preparations of samplesThe g-C 3N 4,WF,PP,stearic acid and PP-g-MA were weighed according to formulations given in Table 1.All of the pure fillers were dried at 85°C in the convection oven for 48h prior to use.Process for preparing WPC based on PP was schematically shown in Fig.1.The mixtures were prepared by using a twin screw extru-der (SHJ-20with average screw diameter of 20mm and average L /D ratio of 40),with a temperature profile of 175/180/185/190/195/195/190°C and a rotating speed of 150rpm,and then theextruded strands were passed through a trough before palletized.Totally 6sets of blends and composite samples were fabricated.The pellets were injected into ISO standard specimens by using an injection molding machine (HMT OENKEY)at 190°C.The actual samples were shown in the Fig.2.2.4.CharacterizationX-ray diffraction (XRD)patterns were recorded on a diffractom-eter (D /max-1200)using graphite monochromatic Cu K a radiation (k =0.1541nm)at a generator voltage of 40kV and a current of 40mA.Measurements were conducted within a 2h range of 2.0–70.0°at a scanning rate of 2°/min.Fourier transform infrared spectrometer (FTIR)spectra between 500and 4000cm À1were obtained on a Nicolet 6700spectrometer (USA).Scanning elec-tronic microscope (SEM)was performed on JSM-6330F scanning electron microscope with an accelerating voltage of 20.0kV.The fracture surfaces of samples were coated with a thin layer of gold before SEM observation.2.5.Water absorption propertiesThe water absorption test was conducted as per ASTM:D570-98.Before testing,five specimens were dried in an oven for 48h at 100±3°C.The weight of dried specimens was measured at an accuracy of 0.001g.The dimensions of samples for water absorption test were 80mm Â10mm Â4mm.The conditioned specimens were placed in a container filled with deionized water,supported on their edges,and entirely immersed and kept at 23±1°C for 16days.At the end of 24±1h,48±1h,96±1h,192±1h,384±1h,the specimens were removed from the water one at a time,all surface water wiped off with a dry cloth and weighed immediately.Five replications were tested and their average values were reported.The value of the water absorption in percentage was calculated by using the following Eq.(1):WA t ð%Þ¼W t ÀW 0W 0Â100ð1Þwhere WA t is the water absorption (%)at time t ,W 0is the oven dried weight and W t is the weight of specimen at a given immersion time.Table 1The blend design of compounding materials for respective composite.Sample PP (wt.%)WF (wt.%)PP-g-MA (wt.%)g-C 3N 4(wt.%)Stearic acid (wt.%)PP 950302WPC5540302WPC/CN 15440312WPC/CN 35240332WPC/CN 55040352WPC/CN 1045403102Fig.1.Scheme of the process for preparing WPC based on PP.104 B.2.6.Mechanical propertiesThe tensile andflexural tests were carried out by using a Universal Testing Machine(LLOYD LR100K)according to the ISO standards527-1[17]and178[18],respectively.The notched Izod impact strengths were conducted following ISO standards179-1 [19]with impact type test machine(ZBC-50).Five replications were tested and their average values were reported.2.7.Thermal propertiesThermal behaviors of WPC and WPC/CN were examined by using a thermo gravimetric analyzer(TGA)to determine the weight loss as a function of temperature.Each composite was heated from30to800°C at a rate of10°C/min under nitrogen atmosphere.3.Results and discussion3.1.XRD analysisFig.3illustrates the X-ray diffraction pattern of the prepared g-size of one tris-s-triazine unit(0.713nm),which could be attrib-uted to the presence of a tilt angularity in the structure[15,16]. The above result indicated that the as-prepared g-C3N4possesseda tri-s-triazine-based structure and the g-C3N4phase was formed.3.2.FTIR spectraFTIR spectra of g-C3N4were shown in Fig.4,which exhibited three characteristic absorption regions located around 3412cmÀ1,1200–1650cmÀ1and799cmÀ1.The adsorption band centered at3412cmÀ1could be ascribed to the stretching mode of OH bond.The broad absorption band at3231cmÀ1could be attributed to the stretching vibration of NH2or N–H groups,sug-gesting the small amount of contamination of hydrogen[21,22]. It was suggested that a small amount of amino groups remained in the samples prepared by direct heating of the melamine.Zhao et al.[23]reported that residual hydrogen atoms bound to the edges of the graphic-like C–N sheet in the form of C–NH2and 2C–NH bonds.Peaks appeared at the range from1241to 1638cmÀ1were attributed to the stretching vibration of graphitic C–N single and double bond characters[24],which again con-firmed the existence of poly(tri-s-triazine)-based p-conjugated systems in g-C3N4heterojunctions.The sharp peak at799cmÀ1 was ascribed to the typical breathing vibration of the triazine units [16,25].The FTIR spectra which obtained confirming that the prod-ucts were the same to the literature treated at550°C under the air atmosphere.3.3.Water absorption propertiesThe water absorption behavior was important to investigation of the durability of the WPC exposing to the environmental condi-tions.The water uptake results of PP,WPC and WPC/CN after immersion in distilled water for sixteen days were shown in Fig.5.As seen,pure PP barely absorbed moisture due to its hydro-phobic nature.However,the amount of water uptake was suddenly increased with the incorporation of WF into PP.So the absorptionFig.2.Images of samples made with different content of g-C3N4.Fig.3.XRD patterns for the synthesized g-C3N4.Fig.4.FTIR spectrum for the synthesized g-C3N4.Design66(2015)103–109105which was even lower than the WPC sample.The reason might be that well dispersity was achieved for1wt.%of g-C3N4which gave better interfacial adhesion of the g-C3N4to the matrix and better water barrier performance[26].According to Das et al.[27],water saturated the cell wall offiber,and then water occupied void spaces.However,when the g-C3N4filler was added from1wt.%to10wt.%,the water uptake of composite was increased,and it was attributed to the agglomerate of g-C3N4that increased voids and crack fractions during the compounding process.This hypoth-esis was also confirmed by SEM images(Fig.7d–f).From which it can be seen that more crack were created when the composite con-tained10wt.%g-C3N4.So the composite would absorb more water in our experimental ranges offillers(more than1wt.%).3.4.SEM analysisFractured surfaces of the composites were obtained by impact fracture at room temperature.Fig.7showed the SEM photographs of the fracture surfaces of the ually some of the stacked plate-shaped g-C3N4would delaminate under plastic shearing process(Fig.6).Thus,the delaminated g-C3N4had higher surface area to be in contact with polymer matrix which it would partly increase the mechanical strength of the composites[28]. Through SEM study,the distribution and compatibility between thefillers and the matrix can be found.Fig.7a showed that the sur-face of the as-prepared g-C3N4was quite smooth and it was in stacked plate shapes.In Fig.7b,the crack could be clearly found between the matrix and the woodfibers.This was attributed to the hydrophilic nature of thefibers and their poor homogeneity in the plastic matrix.Thus,it could be implied that the interfacial interaction between the WF and the PP matrix in the composites was weak,which means thefibers can leave the matrix easily and cavities can also be easily created when stress was applied. Finally,the generated cavities and cracks in WPC would accelerate to water absorption and reduce the mechanical properties.The surface of WPC containing1wt.%g-C3N4analyzed by SEM was depicted in Fig.7c.The stacked plate-shaped g-C3N4was delami-nated under plastic shearing process,and the g-C3N4was well dis-persed in the PP matrix.Thus the1wt.%content of g-C3N4filler showed the maximumflexural strength value which was larger than the other samples,and the decrease in water absorption.This could be further supported by Fig.5.However,Fig.7d showed that the interfacial interaction between g-C3N4and PP matrix was weak,this might due to the hydrophilic nature of the nanofiller, it would easily cause agglomerate when high content of g-C3N4 was rge agglomeration of g-C3N4can be found in Fig.7e and f.Due to the agglomeration,the g-C3N4existed in the form of stack plate but not the delaminated plates.In addition,the agglomeration caused bad interfacial adhesion and more cracks and cavities in the interfacial area.The weak interfacial adhesion hindered stress transferring from the matrix to thefibers which was harmful to the mechanical properties and water absorption of the composite compared with the compositefilled with lower content of g-C3N4.This were further supported by the mechanical tests and water absorption tests.3.5.Mechanical propertiesWhen the woodfiber was added into PP,theflexural and tensile modulus of the composites were increased compared to PP as shown in Table2.This might be attributed to the better stiffness of woodfibers compared with PP.Theflexural and tensile strengths of WPC/CN had the maximum values when the contents of g-C3N4are1wt.%and3wt.%respectively.However,they were decreased when more g-C3N4was added compared with the con-trol sample.As observed from Table2,the composites exhibited a negative elongation at break effect with the addition of g-C3N4. When PPfilled with woodflour show brittle behavior,thus the elongation at break of WPC and WPC/CN decreased markedly com-pared to PP.Fig.8illustrated the effect of g-C3N4content on theflexural properties of WPC/CN.Theflexural strength of the compositesFig.5.Water absorption curves of PP,WPC and WPC/CN.The schematic illustrations of changes of g-C3N4during compoundingprocess.Fig.7.SEM images:(a)g-C3N4,(b)WPC,(c)the fracture surface of WPC/CN1,(d)thefracture surface of WPC/CN3,(e)the fracture surface of WPC/CN5and(f)the fracturesurface of WPC/CN10.Design66(2015)103–109was increased slightly at the1wt.%content of g-C3N4compared to the WPC sample and it began to decrease as more g-C3N4was added.Theflexural strength of the composite mainly depended on the interfacial interaction and the properties of constituents [29,30].The result suggested that the low content of g-C3N4 (1wt.%)had better homogeneous dispersion and better interfacial interactions in the WPC,which enabled effective stress transferring from matrix tofibers and then leading to highflexural strength. But with higher content,the g-C3N4began to agglomerate which resulted in poor dispersion in WPC and then theflexural strength began to decrease,so the composite with10wt.%g-C3N4showed the minimumflexural strength,decreased by7.6%compared the WPC sample.Fig.8showed that theflexural modulus increased with increasing g-C3N4content in the composite,the reason might be that theflexural modulus of g-C3N4was higher than WF.Since theflexural modulus in composite mainly depended on the modu-lus of individual component[33].In addition,plate-shapedfillers had high aspect ratios,and this property increased the wettability of thefillers by the matrix,therefore it was helpful to transfer stress from polymer to the plate-shapedfillers.Fig.9illustrated the effect of g-C3N4content on the tensile properties of WPC/CN.When woodfiber was added,the tensile strength of WPC was decreased compared to pure PP(Table When WF was added in thermoplastics,the tensile strength would be decreased[31].Similar to theflexural strength,the tensile strength of WPC/CN was increased atfirst,tensile strength(3wt.%g-C3N4)among theThen,it was decreased as the g-C3N4wasthat3wt.%g-C3N4had positive effect on tensileg-C3N4reinforced polymer composite.Thisbridge’s function of g-C3N4,which led to betterefficiency from the matrix to thefiller andstrengths.Similar observations of otherPP composites were reported[32].Fig.9alsothat more addition(more than3wt.%)of g-C3N4improve tensile strength.This could be explained again by agglomeration of the g-C3N4.The aggregation of g-C3N4was harm-ful to mechanical properties of the resultant nanocomposites.The tensile modulus of WPC/CN was increased with increasing g-C3N4 content in the composite.But when10wt.%g-C3N4was added into WPC,the tensile modulus was decreased slightly.As it could be seen from Fig.9,with increasing of g-C3N4contents to5wt.%, the tensile modulus value was increased by142.9%,reaching approximately498MPa compared to WPC.This enhancement in tensile modulus might be attributed to the decrease in mobility the polymer chains which might that because the stack state 3N4had restrained the mobility of polymer chains.Fig.10illustrated the Izod impact strengths of the composites made with different content of g-C3N4.The test results showed impact strengths of composite were reduced with increasing content of g-C3N4in WPC.But the impact strengths of the compos-with1wt.%and3wt.%g-C3N4content were almost the same.Table2The mechanical properties of PP,WPC and WPC/CN.Fig.8.Effect of g-C3N4content on theflexural properties of WPC/CN.Fig.9.Effect of g-C3N4content on the tensile properties of WPC/CN.Effect of g-C3N4content on the notched Izod impact strength of WPC/CN.B.Lei et al./Materials and Design66(2015)103–109107The most important reason for the decrease might be that g-C 3N 4platelet restricted the motion of polymer chains and made the composites ually,increasing g-C 3N 4content would constrain ductile deformation of the PP matrix and an increasing proportion of the fracture energy,thus the Izod impact strengths decreased with increasing content of g-C 3N 4in WPC.3.6.Thermal propertiesThe TGA curves were very important to study the thermal prop-erties and degradation behaviors of the composite applied in high temperature.Manufacture of the WPC containing high content of wood fiber at high temperature would degrade the cellulosic mate-rials that led to undesirable effects on the properties of the composite.Fig.11showed the mass loss curves of PP,WPC and WPC/CN.The TGA curve of pure PP showed single-mass loss step from 333°C to 466°C.However,the maximum degradation rate shitted to a higher temperature when PP was mixed with wood fiber,indi-cating that the WF improved the thermal stability of the polymer compared with the pure PP.This could be attributed to the high thermal stability of lignin in WF [33].The cure for WPC mainlyhad two decomposition stages.The first decomposition peak (248–375°C)was mainly due to the thermal decomposition of WF [34],and the second one (406–490°C)was mainly due to ther-mal decomposition of PP (See Table 3).Another important feature was that the addition of g-C 3N 4to the polymer blend clearly increased the degradation temperature of the composite.Obviously,the WPC/CN mainly showed two decompositions stages.The temperature of degradation of the polymer blend with varying amounts of g-C 3N 4increased from 422to 440°C in comparison to WPC sample.Table 4shows the decomposition temperature at different weight loss (T D %)and residual weight (RW%)for WPC and WPC/CN.It was observed that the decomposition temperature values were increased due to the addition of g-C 3N 4into the polymer blend.The 20%weight loss temperature of composite was increased by about 30.5°C when the addition of g-C 3N 4was up to 10wt.%.It was obvious that the decomposition temperatures of 40%,60%and 80%weight loss were all increased when the g-C 3N 4content was increased.But the 40%and 60%weight loss temperatures of composites made scarcely influence between 3wt.%and 5wt.%addition of g-C 3N 4.This might be due to the poor dispersion and agglomeration of g-C 3N 4.The maximum improvement in thermal stability was observed by the inclusion of 10wt.%g-C 3N 4.The RW%value at 600°C for composites was increased with increasing content of g-C 3N 4.This might be attributed to the presence of g-C 3N 4plate which acted as a barrier and delayed the decomposition of volatile products [35].4.ConclusionsThe effects of g-C 3N 4on the physical,mechanical and morpho-logical properties of the WF/PP composites were investigated.The physical and mechanical test results indicated that the properties of the WPC were significantly influenced by the content of g-C 3N 4.The large surface area of g-C 3N 4enhances flexural and tensile modulus of the composites.A suitable content of g-C 3N 4could also improve flexural and tensile strength of the composites.The sample with 1wt.%g-C 3N 4showed lower water absorption.However,the overloaded g-C 3N 4caused the aggregation and then affected the physical and mechanical properties.The addition of g-C 3N 4obviously improved the degradation temperature and the outward color of the WPC.In this study,the addition of g-C 3N 4from 1wt.%to 3wt.%was found to be the optimal condition to prepare WF/PP composite.AcknowledgementsThis work was financially supported by the National Natural Sci-ence Foundation of China (21271087,51172099,11272093and 21006038),the Foundation of Science and Technology Projects of Guangdong Province (2011B010700080),and the 2013Jinan Uni-versity Challenge Cup for Student Extracurricular Academic Science and Technology Work Competition (201312B07and201312B49).Fig.11.TGA curves for PP,WPC and WPC/CN.Table 3The notched Izod impact strengths of PP,WPC and WPC/CN.Samples Impact strengths (kJ/m 2)PP 3.556±0.055WPC3.481±0.227WPC/CN 1 3.295±0.206WPC/CN 3 3.287±0.197WPC/CN 5 3.091±0.163WPC/CN 102.394±0.048Table 4Thermal analysis of WPC and WPC/CN composites.SamplesTemperature of decomposition (T D )in °C at different weight loss (%)RW%at 600°C20%40%60%80%WPC382.36454.36467.46482.1815.01WPC/CN 1383.45457.10470.73488.7316.96WPC/CN 3386.18460.10475.09599.4520.20WPC/CN 5402.55460.9475.63645.8221.50WPC/CN 10412.91465.23480.00670.3625.21Design 66(2015)103–109References[1]Ashori A.Wood–plastic composites as promising green-composites forautomotive industries.Bioresour Technol2008;99:4661–7.[2]Ashori A.Effects of nanoparticles on the mechanical properties of rice straw/polypropylene composites.J Compos Mater2013;47:149–54.[3]Raj RG,Kokta pounding of cellulosefibers with polypropylene:effectoffiber treatment on dispersion in the polymer matrix.J Appl Polym Sci 1989;38:1987–96.[4]Kazayawoko M,Balatinecz JJ,Matuana LM.Surface modification and adhesionmechanisms in woodfiber–polypropylene composites.J Mater Sci 1999;34:6189–99.[5]Bisanda ETN,Ansell MP.The effect of silane treatment on the mechanical andphysical properties of sisal–epoxy pos Sci Technol 1991;41:165–78.[6]Singh B,Gupta M,Verma A.Influence offiber surface treatment on theproperties of sisal–polyester composites.Polym Compos1996;17:910–8. [7]Bakraji EH,Salman N.Properties of wood–plastic composites:effect ofinorganic additives.Radiat Phys Chem2003;66:49–53.[8]Mwaikambo LY,Ansell MP.The effect of chemical treatment on the propertiesof hemp,sisal,jute and kapok for composite reinforcement.Die Angew Makromol Chem1999;272:108–16.[9]Lee SH,Wang S.Biodegradable polymers/bamboofiber biocomposite with bio-based coupling pos Part A–Appl Sci2006;37:80–91.[10]Hosseinaei O,Wang S,Enayati AA,Rials TG.Effects of hemicellulose extractionon properties of woodflour and wood–plastic pos Part A–Appl Sci2012;43:686–94.[11]Ichazo MN,Albano C,Gonzalez J,Perera R,Candal MV.Polypropylenewoodflour composites treatments and pos Struct2001;54: 207–14.[12]Faruk O,Matuana L.Nanoclay reinforced HDPE as a matrix for wood–plasticpos Sci Technol2008;68:2073–7.[13]Younesi KH,Hiziroglu S,Farsi M.Some of the physical and mechanicalproperties of cement composites manufactured from carbon nanotubes and bagassefiber.Mater Des2012;33:395–8.[14]NajafiA,Kord B,Abdi A,Ranaee S.The impact of the nature of nanoclay onphysical and mechanical properties of polypropylene/reedflour nanocomposites.J Thermoplast Compos2011;25:6717–27.[15]Wang XC,Maeda K,Thomas A,Takanabe K,Xin G,Carlsson JM,et al.Polymersemiconductors for artificial photosynthesis:hydrogen evolution by mesoporous graphitic carbon nitride with visible light.J Am Chem Soc 2009;131:1680–1.[16]Yan SC,Li ZS,Zou ZG.Photodegradation performance of g-C3N4fabricated bydirectly heating ngmuir2009;25:10397–401.[17]ISO527-1:2012(E).Plastics-determination of tensile properties-Part1:general principles.[18]ISO178:2001(E).Plastics–determination offlexural properties.[19]ISO179-1:2010(E).Plastics–determination of charpy impact properties–Part1:non-instrumented impact test.[20]Thomas A,Fischer A,Goettmann F,Antonietti M,Müller JO,Schlögl R,et al.Graphitic carbon nitride materials:variation of structure and morphology and their use as metal-free catalysts.J Mater Chem2008;18:4893–908.[21]Li Y,Zhang J,Wang Q,Jin Y,Huang D,Cui Q,et al.Nitrogen-rich carbon nitridehollow vessels:synthesis,characterization,and their properties.J Phys Chem B 2010;114:9429–34.[22]Miller DR,Wang J,Gillan EG.Rapid,facile synthesis of nitrogen-rich carbonnitride powders.J Mater Chem2002;12:2463–9.[23]Zhao Y,Liu Z,Chu W,Song L,Zhang Z,Yu D,et rge-scale synthesis ofnitrogen-rich carbon nitride microfibers by using graphitic carbon nitride as precursor.Adv Mater2008;20:1777–81.[24]Fu J,Chang BB,Tian YL,Xi FN,Dong XP.Novel C3N4–CdS composite photo-catalysts with organic–inorganic heterojunctions:in situ synthesis, exceptional activity,high stability and photocatalytic mechanism.J Mater Chem A2013;1:3083–90.[25]Dong F,Wu LW,Sun YJ,Fu M,Wu ZB,Lee SC.Efficient synthesis of polymeric g-C3N4layered materials as novel efficient visible light driven photo-catalysts.J Mater Chem2011;21:15171–4.[26]Yuan X.Enhanced interfacial interaction for effective reinforcement ofpoly(vinyl alcohol)nanocomposites at low loading of grapheme.Polym Bull 2011;67:1785–97.[27]Das S,Sara AK,Choudhury PK,Basak RK,Mitra BC,Todd T,et al.Effect of steampretreatment of jutefiber on dimensional stability of jute composite.J Appl Polym Sci2000;76:1652–61.[28]Kim HS,Lee BH,Choi SW,Kim S,Kim HJ.The effect of types of maleicanhydride-grafted polypro-pylene(MAPP)on the interfacial adhesion properties of bio-flour-filled polypropylene pos Part A–Appl Sci2007;38:1473–82.[29]Younesi KH,Hiziroglu S,Farsi M.Some of the physical and mechanicalproperties of cement composites manufactured from carbon nanotubes and bagassefiber.Mater Des2012;33:395–8.[30]Kazemi NS,Younesi KH.Effect of sea water on water absorption andflexuralproperties of wood-polypropylene composites.Eur J Wood Wood Prod 2011;69:553–6.[31]Yeh SK,Kim KJ,Gupta RK.Synergistic effect of coupling agents onpolypropylene-based wood–plastic composites.J Appl Polym Sci 2013;127:1047–53.[32]Ashori A,Nourbakhsh A.Preparation and characterization of polypropylene/woodflour/nanoclay composites.Eur J Wood Wood Prod2011;69:663–6. [33]Yildiz S,Gümüs Kaya E.The effects of thermal modification on crystallinestructure of cellulose in soft and hardwood.Build Environ2007;42:62–7. [34]Fisher T,Hajaligol M,Waymack B,Kellogg D.Pyrolysis behavior and kinetics ofbiomass derived materials.J Anal Appl Pyrol2002;2:331–49.[35]Sheshmani S,Amini R.Preparation and characterization of some graphenebased nanocomposite materials.Carbohydr Polym2013;95:348–59.B.Lei et al./Materials and Design66(2015)103–109109。
二维超薄g-C3N4非金属催化材料的制备及其光电催化性能研究
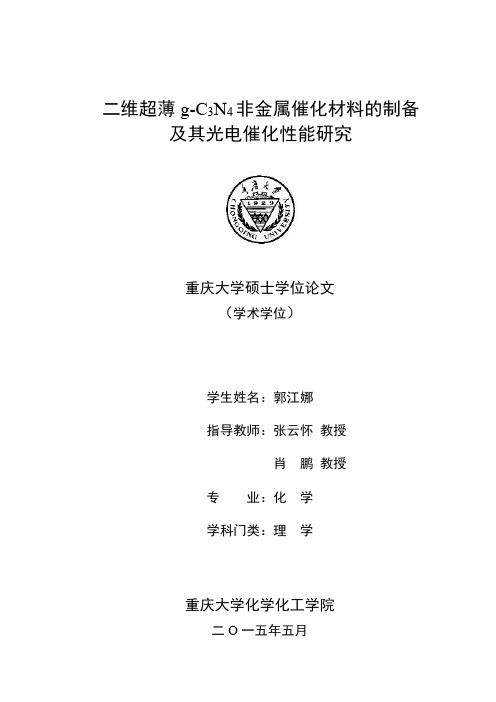
二维超薄g-C3N4非金属催化材料的制备及其光电催化性能研究重庆大学硕士学位论文(学术学位)学生姓名:郭江娜指导教师:张云怀教授肖鹏教授专业:化学学科门类:理学重庆大学化学化工学院二O一五年五月Synthesis and Photoelectrocatalytic Properties of A Metal-free Material: Ultrathin Two-Dimensional Graphitic Carbon Nitride NanosheetsA Thesis Submitted to Chongqing University inPartial Fulfillment of the Requirement for theMaster’s Degree of ScienceByGuo JiangnaSupervised by Prof. Zhang YunhuaiProf. Xiao PengSpecialty: ChemistryCollege of Chemistry and Chemical Engineering of Chongqing University, Chongqing, ChinaMay 2015摘要当今社会,能源危机和环境污染是人类社会持续发展面临的两大挑战,开发清洁的新能源技术刻不容缓。
太阳能具有资源丰富,可循环再生,普遍易得,绿色无公害等诸多优点,而氢作为可再生的二次能源载体,清洁、高效、可贮存、可运输,是理想的绿色能源,因此,利用太阳光分解水产氢是一种储存和利用太阳能的理想途径。
半导体材料是吸收和转化太阳能的关键所在,所以,开发经济高效的光催化剂材料是解决目前太阳能转化利用问题的主要途径之一。
TiO2等传统半导体催化剂还存在可见光利用效率不高,光腐蚀,催化剂稳定性差等问题,这限制了半导体光催化剂的实际应用。
g-C3N4作为一种新型的非金属聚合物半导体,具有二维类石墨烯结构和优越的电子特性,因其较小的带隙能2.7eV,具有可见光响应,能够有效利用太阳能分解水产氢。
层状石墨相g-C3N4氮化碳的简易制备和表征
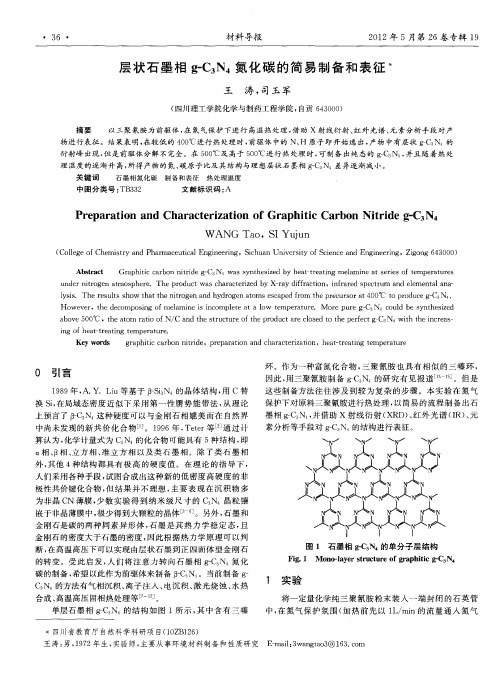
( le e o e s r n a ma e t a g n e i g Co lg fCh mit y a d Ph r c u i l c En i e rn ,S c u n Un v r iy o ce c n g n e i g,Zio g 6 3 0 ih a i e st fS in e a d En i e rn g n 4 0 0) Ab ta t src Gr p i cc r o ir e g C3 s s n h sz d b e kte tn lmi e a e iso e e a u e a h t a b n n ti - wa y t e ie y h a r a i g mea n ts re ft mp r t r s i d N4
0 引 言
18 年 , Y 9 9 A. .Li u等基 于 Js。 3i 一 N 的 晶体 结 构 , c替 用 换 S, i在局 域态 密度 近 似 下 采 用第 一 性 赝 势 能 带法 , 理 论 从 上 预 言 了 Jc N 3 。 这种 硬度 可 以与金 刚石 相媲 美 而 在 自然 界 一
lss y i.Th e ut h w h tt ento e n y rg nao s a e r m h rc ro t4 0 t r d c - . ers lss o t a h i g na d h d o e t mse c p dfo t ep e u s ra 0 ℃ op o u egC3 r N4
i g o e tt e t g t mp r t r . n fh a- r a i e e a u e n
Ke r s y wo d
g a h t a b n n t i e r p r t n a d c a a t rz t n e tte tn e p r t r r p ii c r o i d .p e a a i n h r c e ia i 。h a - r a i g tm e a u e c r o o
gc3n4基光催化剂的制备 -回复
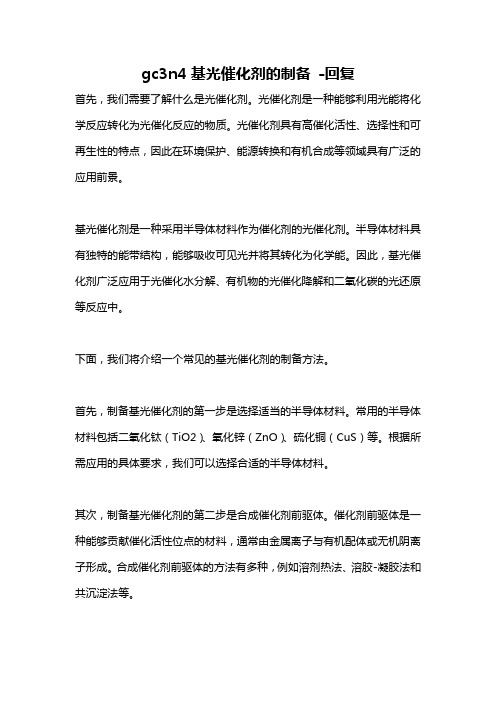
gc3n4基光催化剂的制备-回复首先,我们需要了解什么是光催化剂。
光催化剂是一种能够利用光能将化学反应转化为光催化反应的物质。
光催化剂具有高催化活性、选择性和可再生性的特点,因此在环境保护、能源转换和有机合成等领域具有广泛的应用前景。
基光催化剂是一种采用半导体材料作为催化剂的光催化剂。
半导体材料具有独特的能带结构,能够吸收可见光并将其转化为化学能。
因此,基光催化剂广泛应用于光催化水分解、有机物的光催化降解和二氧化碳的光还原等反应中。
下面,我们将介绍一个常见的基光催化剂的制备方法。
首先,制备基光催化剂的第一步是选择适当的半导体材料。
常用的半导体材料包括二氧化钛(TiO2)、氧化锌(ZnO)、硫化铜(CuS)等。
根据所需应用的具体要求,我们可以选择合适的半导体材料。
其次,制备基光催化剂的第二步是合成催化剂前驱体。
催化剂前驱体是一种能够贡献催化活性位点的材料,通常由金属离子与有机配体或无机阴离子形成。
合成催化剂前驱体的方法有多种,例如溶剂热法、溶胶-凝胶法和共沉淀法等。
接下来,制备基光催化剂的第三步是制备光催化剂。
在这一步中,我们将催化剂前驱体进行适当的处理,以形成具有良好催化性能的光催化剂。
这个过程通常包括固相烧结、溶胶-凝胶法和浸渍法等。
最后,制备基光催化剂的第四步是性能测试和表征。
性能测试是评价光催化剂活性和选择性的关键步骤。
通过一系列的实验测试,如光电化学测试、紫外-可见漫反射光谱(UV-Vis DRS)和气体吸附等,我们可以获得该催化剂的光催化活性、能带结构、表面性质和催化反应机理等关键数据。
综上所述,基光催化剂的制备是一个多步骤的过程。
通过选择适当的半导体材料、合成催化剂前驱体、制备光催化剂以及性能测试和表征,我们可以得到具有高催化活性的基光催化剂。
这种基光催化剂在环境保护、能源转换和有机合成等领域具有广泛的应用前景,对于推动可持续发展具有重要意义。
我们期待着基光催化剂研究的进一步发展,以满足社会的需求。
g-c3n4
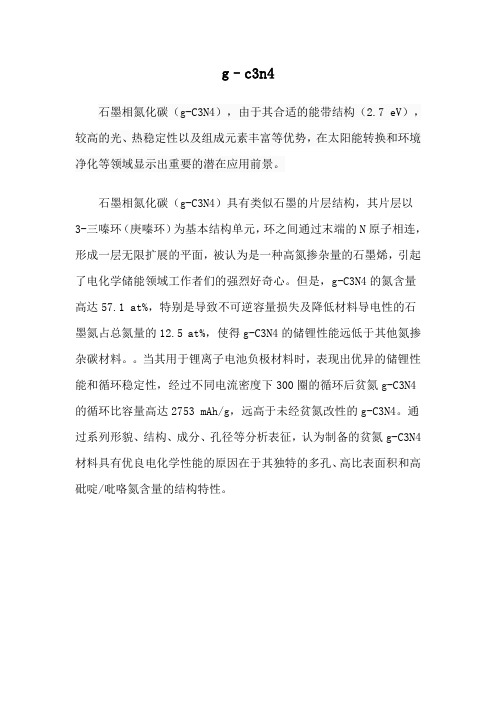
g–c3n4
石墨相氮化碳(g-C3N4),由于其合适的能带结构(2.7 eV),较高的光、热稳定性以及组成元素丰富等优势,在太阳能转换和环境净化等领域显示出重要的潜在应用前景。
石墨相氮化碳(g-C3N4)具有类似石墨的片层结构,其片层以
3-三嗪环(庚嗪环)为基本结构单元,环之间通过末端的N原子相连,形成一层无限扩展的平面,被认为是一种高氮掺杂量的石墨烯,引起了电化学储能领域工作者们的强烈好奇心。
但是,g-C3N4的氮含量高达57.1 at%,特别是导致不可逆容量损失及降低材料导电性的石墨氮占总氮量的12.5 at%,使得g-C3N4的储锂性能远低于其他氮掺杂碳材料。
当其用于锂离子电池负极材料时,表现出优异的储锂性能和循环稳定性,经过不同电流密度下300圈的循环后贫氮g-C3N4的循环比容量高达2753 mAh/g,远高于未经贫氮改性的g-C3N4。
通过系列形貌、结构、成分、孔径等分析表征,认为制备的贫氮g-C3N4材料具有优良电化学性能的原因在于其独特的多孔、高比表面积和高砒啶/吡咯氮含量的结构特性。
g-C3N4固载磷钨酸催化剂的制备及催化性能
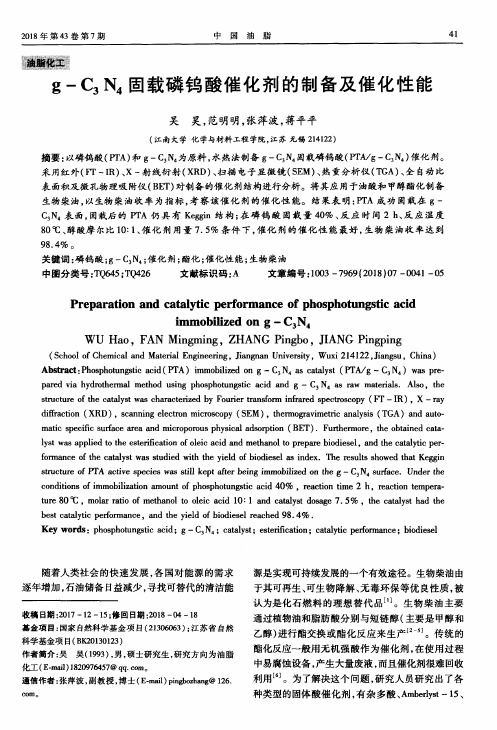
W U Hao,FAN Mingming,ZHANG Pingbo,JIANG Pingping
(School of Chemical and Mater ial Engineer ing,Jiangnan University,Wuxi 214122,Jiangsu,China) Abstract:Phosphotungstie acid(er A)immobilized on g—C3 N4 as catalyst(PTA/g~C3 N4)was pre—
g-C3N4复合TiO2纳米管阵列的制备及表征

· I8 ·
河 南 化 工 HENAN CHEMICAL INDUSTRY
2018年 第 35卷
采 用 尿 素 热 解 法 制 备 g—C N ,控 制 热 解 温 度 为 550℃ ,升 温 速 度 为 3℃/min;然 后 取 适 量 g— C N ,置人 体 积 比 2:1的无水 乙醇 和去 离子 水溶 液 充分 分散 后 制 得 g—C N 悬 浮 液 ;通 过 浸 渍 法 在 TiO 纳米管阵列表面复合 g—C N ,以浸渍的次数 反映 g—C N 的负载 量 。 1.3 样 品表征
第 2期
·开 发 与研 究 ·
周淑慧等 :孚一C,N4复合 TiO:纳米 管阵列的制备及表征
g—C3 N4复 合 TiO2纳 米 管 阵 列 的 制备 及 表 征
周淑慧 ,刘世凯 ,刘鑫鑫
(河南工业大学 材料科 学与工程学 院 ,河南 郑州 450001)
摘 要 :采 用阳极氧化法制备 出 TiO 纳米管 阵列 ,再 通过 浸渍法 实现石墨相 C N (g—C,N )对 TiO 纳米管阵列的 复合 。研 究表明 ,g—C3N4复合能显著提 高 TiO2纳米管 阵列的光 电化 学性 能,且 经三 次 g—C3N4漫渍的 TiO2纳米 管阵列性能 最佳 。 关键 词 :TiO2;纳米管 阵列 ;阳极氧化 ;g—C3N4;光 电化 学性 能 中图分类号 :TQ134.11 文献标识码 :A 文章编号 :1003—3467(2018)02-0017-03
properties
0 引 言
纳米 TiO 具有很高 的化学稳定性 和热稳定性 等优 良性能 ,在太 阳能光转化应 用方面备 受关注。 其中采用阳极氧化法所制备的 TiO 纳米管阵列 (纳 米管阵列)结构有 序规整 ,比表面积大 ,同时又有较 高的量子效率 ,在太 阳能 电池和光催化等方面有广 阔的应用前景 .2 J。但是 由于 TiO:只能利用紫外 光 ,严重阻碍 了 TiO:纳米管阵列在光电转换方面的 应用 ,需 要进 行 进一 步 的改 性 J。围 绕着 这个 问题 国内外进行了大量的研究 ,结果表明半导体 复合是 有效 途 径之 一 。石 墨 相 C,N (g—C N )由于 来 源广 泛 、化学 稳定 性好 及 光 电特性 方 面的优 势 ,被视 为一种半导体复合理 想 的材料 J。本文采 用 阳极
大比表面积介孔g-C3N4材料的制备及其产氢性能

浙江理工大学学报,第49卷,第6期,2023年11月J o u r n a l o f Z h e j i a n g S c i -T e c h U n i v e r s i t yD O I :10.3969/j.i s s n .1673-3851(n ).2023.06.001收稿日期:2023-03-09 网络出版日期:2023-05-05基金项目:国家自然科学基金项目(52003243);浙江省自然科学基金项目(L Q 21E 030010)作者简介:王 聪(1998- ),男,安徽宣城人,硕士研究生,主要从事光催化材料方面的研究㊂通信作者:司银松,E -m a i l :s i ys @z s t u .e d u .c n 大比表面积介孔g -C 3N 4材料的制备及其产氢性能王 聪,颉启东,傅雅琴,司银松(浙江理工大学材料科学与工程学院,杭州310018) 摘 要:为获得大比表面积和优异产氢性能的石墨相氮化碳(g -C 3N 4),将单氰胺和甲醛溶液溶入二氧化硅(S i O 2)溶胶,形成单氰胺㊁甲醛和S i O 2凝胶组成的杂化前驱体,并将该杂化前驱体在通气条件下煅烧以制备介孔g -C 3N 4(命名为V C N ),在密闭条件下煅烧以制备介孔g -C 3N 4(命名为A C N );利用透射电镜㊁X 射线衍射仪㊁傅里叶变换红外光谱等对介孔g -C 3N 4的形貌㊁晶体结构等进行表征,通过荧光光谱和气相色谱仪等分析介孔g -C 3N 4的产氢性能㊂结果表明:V C N 在获得大比表面积(约312m 2/g)的同时,拓宽了光吸收范围(约760n m ),提高了光致电子-空穴对分离效率㊂加入40m g 甲醛的V C N (V C N -40)具有最佳的产氢速率,为2826μm o l /(h ㊃g),比通气条件下未加入甲醛的g -C 3N 4(V C N -0)提高了1.8倍㊂研究结果为开发高产氢效率g -C 3N 4基复合催化剂提供了有益参考㊂关键词:g -C 3N 4;S i O 2溶胶;甲醛;多孔结构;热聚合过程;产氢性能中图分类号:O 643.36文献标志码:A文章编号:1673-3851(2023)11-0665-09引文格式:王聪,颉启东,傅雅琴,等.大比表面积介孔g -C 3N 4材料的制备及其产氢性能[J ].浙江理工大学学报(自然科学),2023,49(6):665-673.R e f e r e n c e F o r m a t :W A N G C o n g ,X I E Q i d o n g ,F U Y a q i n ,e t a l .P r e p a r a t i o n o f m e s o p o r o u s g -C 3N 4ma t e r i a l s w i t h a l a r g e s p e c i f i c s u r f a c e a r e a a n d t h e i r h y d r o g e n p r o d u c t i o n p e r f o r m a n c e [J ].J o u r n a l o f Z h e j i a n g S c i -T e c h U n i v e r s i t y,2023,49(6):665-673.P r e p a r a t i o n o f m e s o p o r o u s g -C 3N 4ma t e r i a l s w i t h a l a r g e s p e c i f i c s u r f a c e a r e a a n d t h e i r h y d r o ge n p r o d u c t i o n p e rf o r m a n c e W A N G C o ng ,X I E Q i d o n g ,F U Y a q i n ,S I Y i n s o n g(S c h o o l o f M a t e r i a l s S c i e n c e &E n g i n e e r i n g ,Z h e j i a n g S c i -T e c h U n i v e r s i t y ,H a n gz h o u 310018,C h i n a ) A b s t r a c t :T o o b t a i n g r a p h i t i c c a r b o n n i t r i d e (g -C 3N 4)wi t h a l a r g e s p e c i f i c s u r f a c e a r e a a n d e x c e l l e n t h y d r o g e n p r o d u c t i o n p r o p e r t y ,t h e s o l u t i o n o f c y a n a m i d e a n d f o r m a l d e h yd e w a s d i s s o l v e d i n t o t h e s i l i c a (S i O 2)s o l ,a n d a h y b r i d p r e c u r s o r c o m p o s e d o f c y a n a m i d e ,f o r m a l d e h y d e a n d S i O 2g e l w a s f o r m e d .M e s o p o r o u s g -C 3N 4o b t a i n e d b y c a l c i n a t i o n o f t h e h y b r i d p r e c u r s o r u n d e r v e n t i l a t i o n c o n d i t i o n (n a m e d V C N )a n d t h a t u n d e r a i r t i g h t c o n d i t i o n (n a m e d A C N )w e r e o b t a i n e d .T h e m o r p h o l o g y a n d c r ys t a l s t r u c t u r e o f m e s o p o r o u s g -C 3N 4we r e c h a r a c t e r i z e d w i t h t r a n s m i s s i o n e l e c t r o n m i c r o s c o p e ,X -r a y d if f r a c t o m e t e r a n d F o u r i e r t r a n s f o r m i n f r a r e d s p e c t r o s c o p y .T h e h y d r og e n p r o d u c t i o n p r o p e r t i e s o f m e s o p o r o u s g -C 3N 4we r e t e s t e d b yf l u o r e s c e n c e s p e c t r a a n dg a s ch r o m a t o g r a p h y .T h e r e s u l t s s h o w t h a t V C N n o t o n l y h a s a l a r g e s p e ci f i c s u r f a c e a r e a (a b o u t 312m 2/g ),b u t a l s o b r o a d e n s t h e l i g h t a b s o r pt i o n r a n g e (a b o u t 760n m )a n d i m p r o v e s t h e s e p a r a t i o n e f f i c i e n c y of t h e p h o t o i n d u c e d e l e c t r o n -h o l e p a i r s .S p e c i f i c a l l y ,V C N w i t h 40mg f o r m a l d eh y d e (V C N -40)p o s s e s s e s t h e hi g h e s t h y d r o g e n p r o d u c t i o n r a t e o f 2826μm o l /(h ㊃g ),w h i c h i s 1.8t i m e s h i g h e r t h a n t h a t o f g -C 3N 4wi t h o u t f o r m a l d e h y d e u n d e r v e n t i l a t i o n c o n d i t i o n (V C N -0).T h i s s t u d y p r o v i d e s u s e f u l r e f e r e n c e f o r t h e d e v e l o p m e n t o f g -C 3N 4-b a s e d c o m p o s i t ec a t a l y s t s w i t h h i g h h yd r o ge n ef f i c i e n c y.K e y w o r d s:g-C3N4;S i O2s o l;f o r m a l d e h y d e;p o r o u s s t r u c t u r e;t h e r m a l p o l y m e r i z a t i o n p r o c e s s;h y d r o g e n p r o d u c t i o n p e r f o r m a n c e0引言面对日益严重的能源危机和环境污染,利用太阳能驱动光催化分解水产氢,在替代传统能源方面极具潜力和优势[1-3]㊂石墨相氮化碳(g-C3N4)因原料丰富㊁易于合成和氧化还原能力较强等优点被广泛用于光催化制氢[4-7],但g-C3N4的光催化活性受到比表面积㊁光致电子-空穴对复合率和可见光吸收能力等限制[8-13]㊂因此,有必要对g-C3N4进行改性以提高其光催化产氢性能㊂多孔材料由于较大的比表面积和可用的多孔结构广泛应用于电子和电化学器件㊁催化剂的载体和多孔无机材料的模板等㊂目前,已有大量有关多孔g-C3N4光催化材料合成和应用的报道㊂如W a n g 等[14]在g-C3N4中引入了介孔结构,其产氢速率在可见光照射下(420n m)达到149μm o l/(h㊃g),相比没有介孔结构的g-C3N4提高了1倍;W u等[15]开发了一种制备多孔g-C3N4纳米管的方法,其产氢速率在可见光照射下(420n m)达到430μm o l/(h㊃g); Y a n g等[16]以单氰胺溶液和硅胶溶液为前驱体,通过溶胶凝胶法合成了新型介孔g-C3N4,表现出较高的比表面积和光催化活性㊂上述方法制备的多孔g-C3N4虽然在一定程度上提高了比表面积和产氢性能,但依旧存在可见光吸收不足㊁光致电子-空穴对分离效率差等问题㊂近年来,利用各种碳质材料改性g-C3N4以提高其可见光吸收能力的研究受到了广泛关注㊂L i 等[17]以少量甲醛改性双氰胺,采用热解法制备新型C/g-C3N4复合材料,该材料显示出比原始g-C3N4更高的光吸收范围和光致电子-空穴对分离效率,在降解污染物方面表现出很高的光催化活性㊂然而,甲醛改性获得的C/g-C3N4复合材料比表面积较低,同时甲醛作为非富氮材料,可能会影响g-C3N4的热聚合过程,对其微观结构造成影响㊂因此,如何在大幅提高g-C3N4比表面积的同时,增强其可见光吸收能力和光致电子-空穴对分离效率,成为进一步提高g-C3N4产氢性能的关键,但对这一问题的研究,目前已有的报道还很少㊂为获得大比表面积和优异产氢性能的g-C3N4,本文通过在二氧化硅(S i O2)的溶胶阶段溶入单氰胺和甲醛溶液,形成单氰胺㊁甲醛和S i O2凝胶杂化前驱体,并调节溶胶凝胶过程中甲醛的引入量和改变杂化前驱体热聚合工艺条件控制热聚合过程中甲醛和单氰胺之间的相互作用,制备出大比表面积介孔结构的g-C3N4㊂通过透射电镜㊁X射线衍射仪和傅里叶变换红外光谱等对介孔g-C3N4的形貌及晶体结构等进行表征,通过荧光光谱和气相色谱仪等分析介孔g-C3N4的产氢性能,探讨甲醛对介孔g-C3N4热聚合过程和产氢性能的影响,为高产氢效率g-C3N4基复合催化剂的开发提供参考㊂1实验部分1.1实验材料正硅酸乙酯㊁异丙醇和三乙醇胺购自上海阿拉丁生化科技有限公司;草酸(95%)㊁氟化氢铵(99.5%)和N a2S O4购自上海麦克林生化科技股份有限公司;甲醛溶液(质量分数18.5%)和无水乙醇购自杭州高晶精细化工有限公司;单氰胺溶液(质量分数50%)购自安徽泽升科技有限公司;氯铂酸溶液(1.34m g/m L)购自南京化学试剂有限公司; N a f i o n溶液(质量分数5%)购自上海S i g m a-A l d r i c h贸易有限公司㊂所用试剂均为分析纯㊂1.2杂化前驱体的制备将20g正硅酸乙酯(T E O S)与10m L超纯水均匀混合,然后加入0.16g草酸,搅拌10h,获得水解液㊂将水解液进行分装,5g每组(共5组),加入1g的单氰胺溶液,其中4组分别加入10㊁20㊁40m g 和80m g的甲醛溶液,然后将5组混合溶液密封并在80ħ下干燥48h,通风80ħ下干燥48h,制得由单氰胺㊁甲醛和S i O2凝胶组成的杂化前驱体㊂1.3介孔g-C3N4光催化剂的制备将制备的5组杂化前驱体分别置于管式炉中,通高纯氮气以排除炉管中的空气,在通氮气30m i n 后,以2ħ/m i n的升温速率加热至550ħ,保温4h;在煅烧后的产物中加入12m L4m o l/L的氟化氢铵,刻蚀24h,然后离心水洗,冻干72h,在80ħ下干燥24h,研磨成粉末,获得的样品根据甲醛加入量分别命名为V C N-0㊁V C N-10㊁V C N-20㊁V C N-40和V C N-80㊂重复1.2的步骤制备5组杂化前驱体,分别置666浙江理工大学学报(自然科学)2023年第49卷于管式炉中,先通氮气30m i n ,接着密闭管式炉并以2ħ/m i n 的升温速率加热至550ħ,保温4h ;煅烧后的产物加入12m L 4m o l /L 的氟化氢铵,刻蚀24h ,然后离心水洗,冻干72h ,在80ħ下干燥24h,研磨成粉末,获得的样品根据甲醛加入量分别命名为A C N -0㊁A C N -10㊁A C N -20㊁A C N -40和A C N -80㊂1.4 光催化产氢实验在300W 氙灯下进行光催化产氢和稳定性测试㊂将20m g 的样品分散在40m L 的去离子水中,超声20m i n ,移入反应容器中,加入10m L 三乙醇胺和0.445m L 氯铂酸溶液,制得反应溶液㊂将反应容器与产氢循环系统连接,以高纯氮气为载体,打开过滤器㊁冷水机和色谱分析仪,将循环系统抽至真空并密闭,然后通过气相色谱分析氢气产量㊂1.5 测试与表征采用扫描电子显微镜(S E M ,H i t a c h i S -4800,日本)和透射电子显微镜(T E M ,J E M -2100,200k V ,日本)观察样品的形貌和结构;采用X 射线衍射仪(X R D ,布鲁克D 8A d v a n c e,德国)分析样品的晶体结构;采用傅里叶变换红外光谱(F T I R ,N i c o l e t I S50,美国)分析样品的内部结构;采用X 射线光电子能谱(X P S ,E S C A L A B X I +,美国)分析样品的元素价态和化学价态;采用紫外分光光度计(日立U H -4150,日本)分析样品的光吸收性能;采用比表面积及孔径分析仪(B e i S h i D e 3H -2000B E T -A ,中国)分析样品的比表面积和孔径分布;采用稳态/瞬态荧光光谱仪(P L ,F L S 1000,英国)分析样品的光激发载流子复合㊂2 结果与讨论2.1 形貌结构分析图1为V C N 和A C N 的扫描电镜图㊂由图1可知,各样品均由粒径均匀的纳米颗粒堆积而成,其间形成了大量孔隙,这主要是使用氟化氢铵去除S i O 2凝胶后造成的㊂图1 V C N 和A C N 的扫描电镜图图2为V C N 和A C N 的透射电镜图㊂由图2可以观察到,各样品均存在明亮且均匀的空心区域,进一步证明了制备的V C N 和A C N 形成了孔隙,该结构有利于提高比表面积,并可为催化反应提供更多的活性位点[18]㊂图2 V C N 和A C N 的透射电镜图采用X 射线衍射对V C N 和A C N 晶体结构进行分析,结果如图3所示㊂通过传统热聚合法制备的块状g -C 3N 4一般在2θ为13.1ʎ和27.4ʎ出现特征峰,其中13.1ʎ的(100)特征峰对应于七嗪单元的766第6期王 聪等:大比表面积介孔g -C 3N 4材料的制备及其产氢性能面内排列,27.4ʎ的(002)特征峰对应于2D 平面层与层之间的堆积[19]㊂由图3(a )可知,V C N 位于13.1ʎ的(100)特征峰消失,这归因于七嗪单元的扭曲或破坏[20];随着甲醛加入量的增加,V C N 位于27.4ʎ的(002)特征峰逐渐增强,表明晶相含量增加,晶相含量越多代表晶体聚合越完整,同时半高宽逐渐变小,表明V C N 的层间排列均匀性提升㊂由于g -C 3N 4的光生电荷传递主要在层间进行,因此层间排列均匀性的提升有利于载流子的传递和产氢反应的顺利进行[21]㊂由图3(b)可知,随着甲醛加入量的增加,A C N 位于27.4ʎ的(002)特征峰逐渐减弱,这表明密闭条件下,甲醛抑制了g -C 3N 4的热聚合过程,使其晶体聚合不完整;A C N 在(002)的峰位置逐渐向左偏移,根据布拉格定律,(002)峰的2θ值越小,表明g -C 3N 4石墨层的平面间距越大㊂图3 V C N 和A C N 的X 射线衍射图图4为V C N 和A C N 的比表面积和孔径分布图㊂块状g -C 3N 4的比表面积约为10m 2/g ,由图4(a )和4(c )可知,V C N 和A C N 比表面积最高达到了312m 2/g ,相对于块状g -C 3N 4,其比表面积得到了显著提升㊂由图4(b )和4(d )可知,V C N 孔径主要分布在8n m 附近,A C N 孔径主要分布在10n m附近,A C N 孔径相比V C N 略有增大,归因于密闭条件下煅烧生成的氨气更有利于拓宽孔径㊂图4 V C N 和A C N 的比表面积和孔径分布图使用傅里叶变换红外光谱表征V C N 和A C N 中的官能团,结果如图5所示㊂由图5可知,V C N 和A C N 的傅里叶变换红外光谱图和块状g -C 3N 4基本一致,证明所制备样品基本结构没有发生明显变化;各样品均在810㊁1700~1200c m -1和3600~3000c m -1处显示出相似的吸收峰,其中在810c m -1的吸收峰是组成g -C 3N 4三嗪单元碳氮环的弯曲振动特征峰[22-23],1700~1200c m -1处的吸866浙江理工大学学报(自然科学)2023年 第49卷收峰是g -C 3N 4碳氮杂环上的C N ㊁C N 和环外C N 伸缩振动特征峰[24-25],3600~3000c m -1处的吸收峰代表g -C 3N 4边缘破损芳香环上 N H 和 N H 2的伸缩振动,或其表面上吸附的水分子的伸缩振动[26];随着甲醛加入量的增加,V C N -40和V C N -80在2177c m -1左右出现吸收峰,其对应于氰基( C N )的不对称拉伸振动,表明当甲醛加入量过多,V C N 会产生氰基缺陷,过多的氰基缺陷会限制V C N 的光催化性能[27]㊂而在密闭条件下加入甲醛后,A C N 同样产生了氰基缺陷,这不利于提升其光催化性能㊂图5 V C N 和A C N 的傅里叶变换红外光谱图使用X 射线光电子能谱进一步表征V C N 表面官能团情况,结果如图6所示㊂由图6(a)可知,V C N 主要由元素C ㊁N 和O 组成㊂随着甲醛加入量的增加,V C N 的O 含量逐渐降低,C 和N 含量逐渐增加,这是因为在杂化前驱体制备过程中,甲醛中的 C H O 和单氰胺中的 N H 2通过醛胺缩合反应形成交联键,使单氰胺结构更加稳定,从而有效减少了单氰胺在热聚合过程中的损失率[28]㊂由图6(b )可知,通过拟合得到了284.8㊁286.4e V 和288.4e V 三个峰,分别归属于C C 键中的杂质碳或碳原子㊁C N 芳香环中的碳原子[29]和N C N 键的s p 2杂化碳原子[30]㊂随着甲醛加入量的增加,C 含量不断提升,V C N 位于284.8e V 和288.4e V的峰强逐渐增大㊂同时V C N 三个峰的峰位置逐渐向左偏移,这是因为甲醛引起了V C N 电子云密度的变化㊂由图6(c )可知,通过拟合得到了398.8㊁400.0e V 和401.2e V 三个峰,它们分别归属于三嗪单元中C N C ㊁N (C )3和 N H 2基团中的氮原子[31-32]㊂随着甲醛加入量增加,V C N 位于398.8e V 和400e V 的峰强逐渐增大,这是N 的相对含量不断增加导致的㊂此外,V C N -80位于401.2e V 的峰强相比其他V C N 显著增大,表面氨基增多,这归因于其晶体聚合不完全㊂图6 V C N 的X 射线光电子能谱图A C N 的X 射线光电子能谱图如图7所示㊂由图7(a )可知,随着甲醛加入量的增加,A C N 的C ㊁N 含量略微下降㊂这是因为在高温下,甲醛与单氰胺形成的交联键断开后重新释放甲醛,但甲醛无法排出密闭的管式炉而滞留在管式炉内,与部分中间体(三聚氰胺㊁蜜白胺和蜜勒胺等[33])发生反应,因而影响了g -C 3N 4的热聚合过程㊂从图7(b ) (c )中可以看出,随着甲醛加入量的增加,A C N 位于288.4㊁398.8e V 和400.0e V 的峰强均逐渐降低,表明C 和N 的相对含量不断减少,这进一步表明甲醛在密闭条件下会影响A C N 的热聚合过程㊂966第6期王 聪等:大比表面积介孔g -C 3N 4材料的制备及其产氢性能图7 A C N 的X 射线光电子能谱图2.2 光学性能分析 图8为V C N 和A C N 的光学照片㊂由图8可知,V C N 和A C N 的颜色随着甲醛加入量的增加逐渐加深,并向深棕色转变㊂颜色越深代表着光吸收能力越强㊂图8 V C N 和A C N 的光学照片为了验证上述结论,测试了V C N 和A C N 的光学性能,V C N 和A C N 的紫外吸收光谱图如图9所示㊂由图9可知,随着甲醛加入量的增加,各样品在400~800n m 的光吸收范围不断扩展,其中V C N从600n m 扩展到760n m ㊂表明V C N 和A C N 光吸收能力显著提高㊂通过式(1)进一步计算其禁带宽度:E g =1240/λg(1)其中:E g 为禁带宽度,λg为吸收阈值㊂通过计算得出,V C N 和A C N 的带隙均随着甲醛加入量的增加逐渐降低㊂理论上带隙越低,越利于电子-空穴对复合,其中V C N -80的带隙降至1.63e V ,当带隙过于低时,g -C 3N 4氧化还原能力减弱,不利于光催化活性提升㊂电荷载体的分离对增强g -C 3N 4的光催化活性也起着至关重要的作用㊂因此,使用稳态荧光光谱表征光致电子-空穴的复合情况,分析光激发载流子的电荷迁移㊁转移和分离过程㊂由图10可知,随着甲醛加入量的逐渐增加,V C N 和A C N 的峰强度逐渐降低,表明光激发载流子的结合受到甲醛的显著抑制,将有利于光催化活性的提升[34]㊂2.3 产氢性能分析图11为V C N 和A C N 的产氢性能图㊂由图11(a ) (b )可知,加入甲醛后,V C N 产氢速率得到显著提升,且随着甲醛加入量的增加呈递增趋势㊂其中V C N -40产氢速率达到2826μm o l /(h ㊃g ),是V C N -0的1.8倍,这主要是因为随着甲醛加入量的增加,V C N 在保持高比表面积的同时层间排列更均匀㊁光吸收范围更广且光致电子-空穴对分离效率更高㊂与V C N -40相比,V C N -80层间排列均匀性和光致电子-空穴对分离效率的提升并不明显且产生了更多的氰基缺陷,其过低的带隙降低了氧化还原能力,导致了V C N -80产氢速率的下降㊂在大多数实际应用中,产氢稳定性也是一个重要因素㊂因此本文对性能最优的V C N -40进行了16h 循环稳定性测试㊂由图11(c )可知,所制备的V C N -40具有稳定的产氢性能㊂密闭条件下A C N 的产氢性能如图11(d ) (e )所示,其产氢速率随着甲醛加入量的增加逐渐下降,这是由于密闭条件下的甲醛与部分中间体反应影响了g -C 3N 4的热聚合过程,使其聚合不完整,因此产氢性能下降㊂76浙江理工大学学报(自然科学)2023年 第49卷图9 V C N 和A C N 的紫外吸收光谱图图10 V C N 和A C N 的稳态荧光光谱图图11 V C N 和A C N 的产氢性能图为验证本文制备的介孔g -C 3N 4产氢性能,将其与国内外报道的典型多孔g -C 3N 4的产氢性能进行对比㊂不同多孔g -C 3N 4产氢性能如表1所示,相比已报道的其他方法所制备多孔结构g -C 3N 4,V C N -40的产氢性能有一定提升㊂176第6期王 聪等:大比表面积介孔g -C 3N 4材料的制备及其产氢性能表1 不同多孔g -C 3N 4的产氢性能光催化剂光照条件助催化剂产氢速率/(μm o l ㊃h -1㊃g -1)参考文献V C N -0λ>400n m3%P t1609本文V C N -40λ>400n m3%P t2826本文多孔g -C 3N 4纳米片λ>400n m1%P t1395文献[35]多孔g -C 3N 4纳米管λ>420n m3%P t430文献[15]多孔g -C 3N 4λ>420n m3%P t1994文献[36]氧掺杂多孔g -C 3N 4λ>420n m3%P t1968文献[37]3 结 论本文在S i O 2的溶胶阶段加入单氰胺和甲醛溶液,形成单氰胺㊁甲醛和S i O 2凝胶杂化前驱体,并在通气条件下煅烧制备V C N ,在密闭条件下煅烧制备A C N ,分析甲醛对介孔g -C 3N 4热聚合过程及产氢性能的影响,主要结论如下:a )通气条件下,随着甲醛加入量的增加,V C N 晶体聚合更加完整,层间排列均匀性得到提升;密闭条件下,随着甲醛加入量的增加,A C N 聚合不完整,且产生了氰基缺陷㊂因此,甲醛在不同煅烧环境下对介孔g -C 3N 4热聚合过程影响是不同的㊂b )V C N 呈现均匀的介孔结构,具备大比表面积(约312m 2/g )的同时,拓宽了光吸收范围(约760n m ),提高了光致电子-空穴对分离效率㊂c )V C N 的产氢性能得到显著提升,其中加入40m g 甲醛的V C N 具有最佳的产氢性能(2826μm o l /(h ㊃g )),是未加入甲醛的g -C 3N 4的1.8倍㊂参考文献:[1]章冰莹,吕汪洋.g -C 3N 4/T i O 2活化过一硫酸氢盐光催化降解卡马西平的性能[J ].浙江理工大学学报(自然科学版),2022,47(6):873-881.[2]李帅帅,汪星,李敏,等.三维自支撑N i 2P 纳米片阵列的制备及析氢性能[J ].浙江理工大学学报(自然科学版),2020,43(1):32-38.[3]廖金龙,朱曜峰.g -C 3N 4/N a T a O 3复合材料的制备及可见光催化性能[J ].浙江理工大学学报(自然科学版),2019,41(3):319-326.[4]J i a n g J J ,Z h a n g F J ,W a n g Y R .Re v i e w of d i f f e r e n t s e r i e s o f M O F /g -C 3N 4co m p o s i t e s f o r p h o t o c a t a l y t i c h y d r o g e n p r o d u c t i o n a n d C O 2re d u c t i o n [J ].N e w J o u r n a l of C h e m i s t r y,2023,47(4):1599-1609.[5]C h e n X J ,S h i R ,C h e n Q ,e t a l .T h r e e -d i m e n s i o n a lp o r o u s g -C 3N 4fo r h i g h l y e f f i c i e n t p h o t o c a t a l y t i c o v e r a l l w a t e r s p l i t t i n g [J ].N a n o E n e r g y,2019,59:644-650.[6]L i a o G F ,G o n g Y ,Z h a n g L ,et a l .S e m i c o n d u c t o r p o l y m e r i c g r a p h i t i c c a r b o n n i t r i d e p h o t o c a t a l ys t s :t h e h o l y g r a i l f o r t h e p h o t o c a t a l y t i c h y d r o ge n e v o l u t i o n r e a c t i o n u n d e r v i s i b l e l i g h t [J ].E n e r g y &E n v i r o n m e n t a l S c i e n c e ,2019,12(7):2080-2147.[7]黄倩,吕汪洋.二维氮化碳载铜聚酰胺纤维的制备及其抗菌性能[J ].浙江理工大学学报(自然科学版),2022,47(4):482-489.[8]王纯,吕汪洋.石墨相氮化碳负载钴酞菁光催化还原二氧化碳的性能分析[J ].浙江理工大学学报(自然科学版),2021,45(4):462-469.[9]闫玉梅,钱德桂,崔灿.g -C 3N 4/T i O 2异质结光催化材料的制备及光解水产氢性能[J ].浙江理工大学学报(自然科学版),2019,41(6):755-762.[10]张超,汪建新,杨万丽.一维/二维P A N I /g -C 3N 4复合材料的制备及其光催化性能研究[J ].广东化工,2023,50(2):7-10.[11]张称称,李楠,吕汪洋,等.类石墨相C 3N 4的制备及其光催化降解酸性红G 的研究[J ].浙江理工大学学报(自然科学版),2014,31(3):117-121.[12]徐泽忠,高雅,龚俊杰,等.Z n O /g -C 3N 4复合物制备及其光催化降解罗丹明B 性能[J ].哈尔滨商业大学学报(自然科学版),2023,39(1):25-31.[13]钱周琦,杜晓琳,刘琳.掺B r 氮化碳-纤维素复合材料的制备及其对亚甲基蓝的光催化降解性能[J ].浙江理工大学学报(自然科学版),2018,39(6):686-691.[14]W a n g X C ,M a e d a K ,C h e n X F ,e t a l .P o l ym e r s e m i c o n d u c t o r s f o r a r t i f i c i a l p h o t o s y n t h e s i s :h y d r o ge n e v o l u t i o n b y m e s o p o r o u s g r a ph i t i c c a r b o n n i t r i d e w i t h v i s i b l e l i gh t [J ].J o u r n a l o f t h e A m e r i c a n C h e m i c a l S o c i e t y,2009,131(5):1680-1681.[15]W u M ,Z h a n gJ ,H e B B ,e t a l .I n -s i t u c o n s t r u c t i o n o f c o r a l -l i k e p o r o u s P -d o p e d g -C 3N 4tu b e s w i t h h y b r i d 1D /2D a r c h i t e c t u r e a n d h i g h e f f i c i e n t p h o t o c a t a l yt i c h y d r o g e n e v o l u t i o n [J ].A p p l i e d C a t a l ys i s B :E n v i r o n m e n t a l ,2019,241:159-166.[16]Y a n g S Y ,Z h o u W Y ,G e C Y ,e t a l .M e s o po r o u s p o l y m e r i c s e m i c o n d u c t o r m a t e r i a l s o f g r a p h i t i c -C 3N 4:g e n e r a l a n d e f f i c i e n t s y n t h e s i s a n d t h e i r i n t e gr a t i o n w i t h s y n e r g i s t i c A g B r N P s f o r e n h a n c e d p h o t o c a t a l yt i c 276浙江理工大学学报(自然科学)2023年 第49卷p e r f o r m a n c e s[J].R S C A d v a n c e s,2013,3(16):5631-5638.[17]L i Y,J i C N,L u Y X,e t a l.I n s i t u s y n t h e s i s o fc a r b o n/g-C3N4c o m p o s i t e s f o r v i s i b l e l i g h t c a t a l y s i s b y f a c i l e o n e-s t e p p y r o l y s i s o f p a r t i a l l y f o r m a lde h y d e-m o d if i e d d i c y a n d i a m i d e[J].M a t e r i a l s C h e m i s t r y a n d P h y s i c s,2018,214:28-33.[18]W o n J H,K i m M K,O h H S,e t a l.S c a l a b l e p r o d u c t i o n o f v i s i b l e l i g h t p h o t o c a t a l y s t s w i t h e x t e n d e d n a n o j u n c t i o n s o f W O3/g-C3N4u s i n g z e t a p o t e n t i a l a n d p h a s e c o n t r o l i n s o l-g e l p r o c e s s[J].A p p l i e d S u r f a c e S c i e n c e,2023,612:155838.[19]L u o W J,C h e n X J,W e i Z,e t a l.T h r e e-d i m e n s i o n a l n e t w o r k s t r u c t u r e a s s e m b l e d b y g-C3N4n a n o r o d s f o r i m p r o v i n g v i s i b l e-l i g h t p h o t o c a t a l y t i c p e r f o r m a n c e[J].A p p l i e d C a t a l y s i s B:E n v i r o n m e n t a l,2019,255: 117761.[20]Y a n J,R o d r i g u e s M F,S o n g Z L,e t a l.R e v e r s i b l ef o r m a t i o n o f g-C3N43D h y d r og e l s th r o u g hi o n i c l i q u i d a c t i v a t i o n:g e l a t i o n b e h a v i o r a n d r o o m-t e m p e r a t u r e g a s-s e n s i n g p r o p e r t i e s[J].A d v a n c e d F u n c t i o n a l M a t e r i a l s,2017,27(22):1700653.[21]X i a Y Q,Z h a n g N G,Z h o u Z P,e t a l.I n c o r p o r a t i n g S i O2f u n c t i o n a l i z e d g-C3N4s h e e t s t o e n h a n c e a n t i c o r r o s i o n p e r f o r m a n c e o f w a t e r b o r n e e p o x y[J]. P r o g r e s s i n O r g a n i c C o a t i n g s,2020,147:105768.[22]T a n L,Y u C F,W a n g M,e t a l.S y n e r g i s t i c e f f e c t o fa d s o r p t i o n a n d p h o t o c a t a l y s i s o f3D g-C3N4-a g a r h yb r i d a e r o g e l s[J].A p p l i e d S u r f ac e S c i e n c e,2019,467/468: 286-292.[23]Y a n g Z,Y a n J,L i a n J B,e t a l.g-C3N4/T i O2 n a n o c o m p o s i t e s f o r d e g r a d a t i o n o f c i p r o f l o x a c i n u n d e r v i s i b l e l i g h t i r r a d i a t i o n[J].C h e m i s t r y S e l e c t,2016,1 (18):5679-5685.[24]H u a n g S Q,X u Y G,G e F Y,e t a l.T a i l o r i n g o fc r y s t a l l i n e s t r u c t u r e o f c a r b o n n i t r idef o r s u p e r i o r p h o t o c a t a l y t i c h y d r og e n e v o l u t i o n[J].J o u r n a l o fC o l l o i d a n d I n t e r f a c e S c i e n c e,2019,556:324-334.[25]D e n g P,L i u Y Y,S h i L,e t a l.E n h a n c e d v i s i b l e-l i g h t H2e v o l u t i o n p e r f o r m a n c e o f n i t r o g e n v a c a n c y c a r b o n n i t r i d e b y i m p r o v i n g c r y s t a l l i n i t y[J].O p t i c a l M a t e r i a l s,2021,120:111407.[26]王寅,类淑来,张欣,等.碳氮缺陷修饰g-C3N4/W S2异质结光催化性质的第一性原理研究[J].原子与分子物理学报,2023,40(3):25-32.[27]S i Y S,S u n Z Z,H u a n g L M,e t a l.A s h i p-i n-a-b o t t l e s t r a t e g y t o f a b r ic a t e h i g h l y c r y s t a l l i z ed n a n o p o r o u s g r a p h i t i c C3N4m i c r o s p he r e s u n d e r p r e s s u r i z e d c o n d i t i o n s[J].J o u r n a l of M a t e r i a l sC h e m i s t r y A,2019,7(15):8952-8959.[28]W a n g X N,H a n D Y,D i n g Y,e t a l.A l o w-c o s t a n dh i g h-y i e l d a p p r o a c h f o r p r e p a r i n g g-C3N4w i t h a l a r g e s p e c i f i c s u r f a c e a r e a a n d e n h a n c e d p h o t o c a t a l y t i c a c t i v i t y b y u s i n g f o r m a l d e h y d e-t r e a t e d m e l a m i n e[J]. J o u r n a l o f A l l o y s a n d C o m p o u n d s,2020,845:156293.[29]王莹莹,王海花,马永宁,等.基于气体模板法制备的多孔g-C3N4对罗丹明B的降解效果及机理[J].环境工程学报,2022,16(10):3243-3254. [30]马帅帅,顾建东,高媛,等.多孔超薄g-C3N4纳米片负载P t复合材料的制备及其光催化性能[J].无机化学学报,2021,37(8):1439-1448.[31]F e i B,T a n g Y W,W a n g X Y,e t a l.O n e-p o t s y n t h e s i s o f p o r o u s g-C3N4n a n o m a t e r i a l s w i t h d i f f e r e n t m o r p h o l o g i e s a n d t h e i r s u p e r i o r p h o t o c a t a l y t i c p e r f o r m a n c e[J].M a t e r i a l s R e s e a r c h B u l l e t i n,2018, 102:209-217.[32]X u J,Z h a n g L W,S h i R,e t a l.C h e m i c a l e x f o l i a t i o n o fg r a p h i t i c c a r b o n n i t r i d e f o r e f f i c i e n t h e t e r o g e n e o u s p h o t o c a t a l y s i s[J].J o u r n a l o f M a t e r i a l s C h e m i s t r y A, 2013,46:14766-14772.[33]K e s s l e r F K,Z h e n g Y,S c h w a r z D,e t a l.F u n c t i o n a lc a r b o n n i t r ide m a t e r i a l s-d e s i g n s t r a t e g i e sf o r e l e c t r o c h e m i c a l d e v i c e s[J].N a t u r e R e v i e w s M a t e r i a l s, 2017,2(6):17030.[34]李守博,赵蕊霞,胡俊,等.以氰胺废渣为模板制备多孔g-C3N4及其催化性能研究[J].四川化工,2019,22 (5):1-5.[35]T o n g J C,Z h a n g L,L i F,e t a l.A n e f f i c i e n t t o p-d o w na p p r o a c h f o r t h e f ab r ic a t i o n o f l a r g e-a s p e c t-r a t i o g-C3N4n a n o s h e e t s w i t h e n h a n c ed p h o t o c a t a l y t i c a c t i v i t ie s [J].P h y s i c a l C h e m i s t r y C h e m i c a l P h y s i c s,2015,17: 23532-23537.[36]L i K,X i e X,Z h a n g W D.P o r o u s g r a p h i t i c c a r b o n n i t r i d e d e r i v e d f r o m m e l a m i n e-a m m o n i u m o x a l a t e s t a c k i n g s h e e t s w i t h e x c e l l e n t p h o t o c a t a l y t i c h y d r o g e n e v o l u t i o n a c t i v i t y[J].C h e m C a t C h e m,2016,8(12): 2128-2135.[37]Z h a n g J W,G o n g S,M a h m o o d N,e t a l.O x y g e n-d o p e d n a n o p o r o u s c a r b o n n i t r i d e v i a w a t e r-b a s e d h o m o g e n e o u s s u p r a m o l e c u l a r a s s e m b l y f o r p h o t o c a t a l y t i c h y d r o g e n e v o l u t i o n[J].A p p l i e d C a t a l y s i s B:E n v i r o n m e n t a l,2018, 221:9-16.(责任编辑:张会巍)376第6期王聪等:大比表面积介孔g-C3N4材料的制备及其产氢性能。
g-c3n4纳米片的合成及可见光催化降解亚甲基蓝
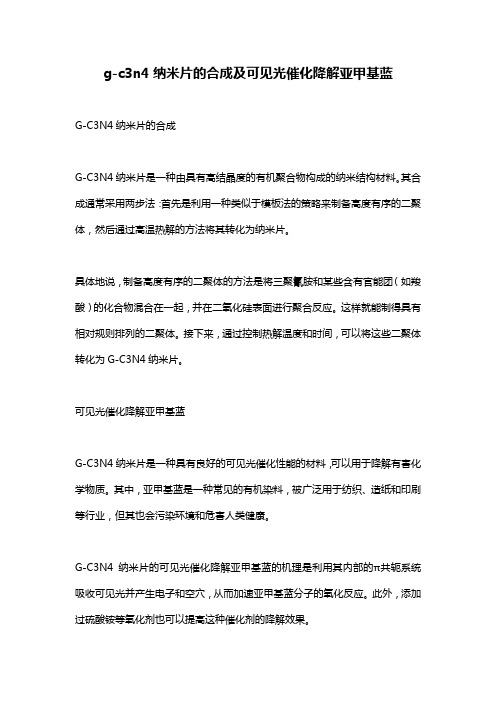
g-c3n4纳米片的合成及可见光催化降解亚甲基蓝
G-C3N4纳米片的合成
G-C3N4纳米片是一种由具有高结晶度的有机聚合物构成的纳米结构材料。
其合成通常采用两步法:首先是利用一种类似于模板法的策略来制备高度有序的二聚体,然后通过高温热解的方法将其转化为纳米片。
具体地说,制备高度有序的二聚体的方法是将三聚氰胺和某些含有官能团(如羧酸)的化合物混合在一起,并在二氧化硅表面进行聚合反应。
这样就能制得具有相对规则排列的二聚体。
接下来,通过控制热解温度和时间,可以将这些二聚体转化为G-C3N4纳米片。
可见光催化降解亚甲基蓝
G-C3N4纳米片是一种具有良好的可见光催化性能的材料,可以用于降解有害化学物质。
其中,亚甲基蓝是一种常见的有机染料,被广泛用于纺织、造纸和印刷等行业,但其也会污染环境和危害人类健康。
G-C3N4纳米片的可见光催化降解亚甲基蓝的机理是利用其内部的π共轭系统吸收可见光并产生电子和空穴,从而加速亚甲基蓝分子的氧化反应。
此外,添加过硫酸铵等氧化剂也可以提高这种催化剂的降解效果。
总的来说,G-C3N4纳米片是一种具有重要应用潜力的纳米材料,在环境治理和能源领域方面具有广泛的应用前景。
g-C3N4基光催化剂的制备及性能的研究

g-C3N4基光催化剂的制备及性能的研究g-C3N4基光催化剂的制备及性能的研究近年来,随着环境污染和能源危机的日益加剧,人们对环境友好和高效能源转化技术的需求与日俱增。
因此,研究开发一种高效的光催化剂成为了科学家们的研究焦点之一。
g-C3N4(石墨相氮化碳)因其良好的光催化性能而备受关注,可以用来降解有害物质、产生氢气或二氧化碳还原等。
g-C3N4基光催化剂的制备方法主要有热处理法、因子法、溶胶-凝胶法、冷冻干燥法等。
其中,热处理法是制备g-C3N4最常见的方法之一。
首先,选择适当和廉价的前驱体(如尿素),通过热聚合反应形成含有大量三氮(C3N4)键的大分子聚合物。
然后,将聚合物在高温条件下进行热分解,生成g-C3N4晶体。
这种方法具有简单、经济的优势,但由于热解条件的限制,得到的g-C3N4往往具有较低的比表面积和较大的颗粒粒径。
研究者们还通过导入不同的掺杂元素,如硫、铁和硼等,来改善g-C3N4的光催化性能。
掺杂能够引入新的能带,扩宽g-C3N4的光响应范围,增强电子和空穴的分离效率。
此外,选择合适的载体材料也可以提高g-C3N4的催化活性。
由于g-C3N4的特殊结构,它在水中的分散性较差。
因此,在制备g-C3N4光催化剂时,通常采用混合材料的形式,例如将g-C3N4与TiO2、ZnO或二氧化硅等载体相结合。
这些载体材料可以提供更大的比表面积和更好的分散性,从而促进光催化剂的光吸收和催化反应效率。
除了制备方法的改进,研究者们还注意到了g-C3N4的光催化机理和提高催化性能的策略。
g-C3N4在光照条件下可以产生电子-空穴对,其中电子和空穴分别参与催化反应中的还原和氧化过程。
因此,提高g-C3N4的分离效率是提高光催化性能的关键。
研究发现,调控杂质浓度和晶体结构可以有效地改善g-C3N4光生载流子的分离效率。
此外,结构修饰和表面修饰也是提高g-C3N4光催化活性的重要途径。
例如,g-C3N4的表面可通过改性和修饰来引入更多的催化活性位点,提高催化剂的催化活性和稳定性。
g-C3N4的制备及可见光催化性能研究
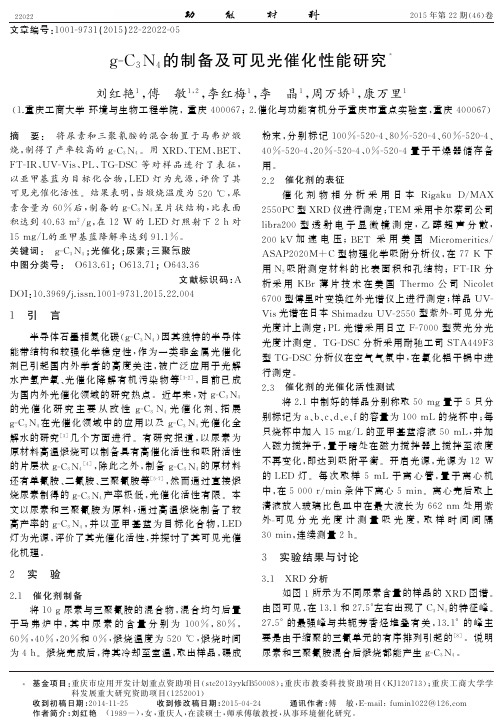
摘 要: 将 尿 素 和 三 聚 氰 胺 的 混 合 物 置 于 马 弗 炉 煅 烧,制得了产率较高的 gGC3N4.用 XRD、TEM、BET、 FTGIR、UVGVis、PL、TGGDSC 等 对 样 品 进 行 了 表 征, 以亚甲基蓝 为 目 标 化 合 物,LED 灯 为 光 源,评 价 了 其 可见光催化活性.结果表明,当煅烧温度为520 ℃,尿 素含量 为 60% 后,制 备 的 gGC3N4呈 片 状 结 构,比 表 面 积达到40.63 m2/g,在12 W 的 LED 灯 照 射 下 2h 对 15 mg/L的亚甲基蓝降解率达到91.1%. 关 键 词 : gGC3N4;光 催 化 ;尿 素 ;三 聚 氰 胺 中 图 分 类 号 : O613.61;O613.71;O643.36
3 实 验 结 果 与 讨 论
3.1 XRD 分析 如图1所示为不同尿素含量的样品的 XRD 图谱.
由 图可见,在13.1和27.5°左右出现了 C3N4的特征峰. 27.5°的最强峰与 共 轭 芳 香 烃 堆 叠 有 关,13.1° 的 峰 主 要是由于缩聚的三氰单元的有序 排 列 引 起 的 . [8] 说 明 尿素和三聚氰胺混合后煅烧都能产生 gGC3N4.
22022
文 章 编 号 :1001G9731(2015)22G22022G05
2015 年 第 22 期 (46)卷
gGC3N4的2,李 红 梅1,李 晶1,周 万 娇1,康 万 里1
(1.重庆工商大学 环境与生物工程学院,重庆 400067;2.催化与功能有机分子重庆市重点实验室,重庆 400067)
2 实 验
2.1 催 化 剂 制 备 将10g尿素与三聚氰胺的 混 合 物,混 合 均 匀 后 置
gc3n4基光催化剂的制备
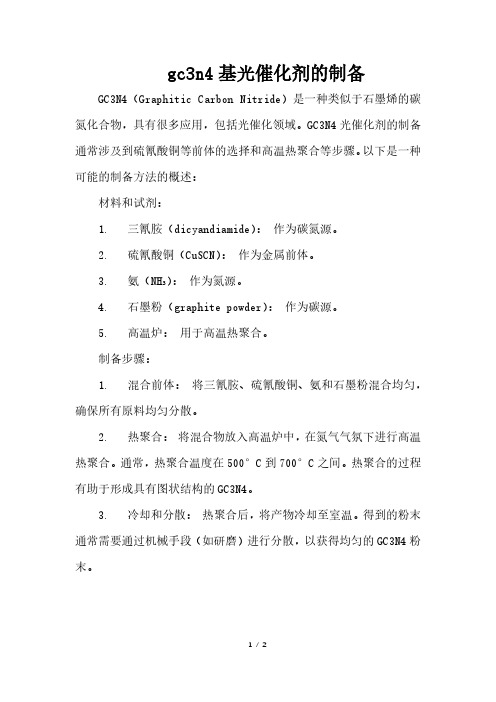
gc3n4基光催化剂的制备GC3N4(Graphitic Carbon Nitride)是一种类似于石墨烯的碳氮化合物,具有很多应用,包括光催化领域。
GC3N4光催化剂的制备通常涉及到硫氰酸铜等前体的选择和高温热聚合等步骤。
以下是一种可能的制备方法的概述:
材料和试剂:
1. 三氰胺(dicyandiamide):作为碳氮源。
2. 硫氰酸铜(CuSCN):作为金属前体。
3. 氨(NH₃):作为氮源。
4. 石墨粉(graphite powder):作为碳源。
5. 高温炉:用于高温热聚合。
制备步骤:
1. 混合前体:将三氰胺、硫氰酸铜、氨和石墨粉混合均匀,确保所有原料均匀分散。
2. 热聚合:将混合物放入高温炉中,在氮气气氛下进行高温热聚合。
通常,热聚合温度在500°C到700°C之间。
热聚合的过程有助于形成具有图状结构的GC3N4。
3. 冷却和分散:热聚合后,将产物冷却至室温。
得到的粉末通常需要通过机械手段(如研磨)进行分散,以获得均匀的GC3N4粉末。
4. 表征:使用各种分析技术,如X射线衍射(XRD)、透射电镜(TEM)、红外光谱(FTIR)等,对制备得到的GC3N4进行表征,以确认其结构和性质。
5. 应用:制备得到的GC3N4可以用作光催化剂,例如在水分解、二氧化碳还原等反应中表现出良好的光催化性能。
请注意,具体的制备条件和步骤可能会因研究的具体要求而有所不同。
在进行实验时,请参考相关文献和研究论文,以确保采用适当的制备方法。
g-c3n4纳米片的合成及可见光催化降解亚甲基蓝
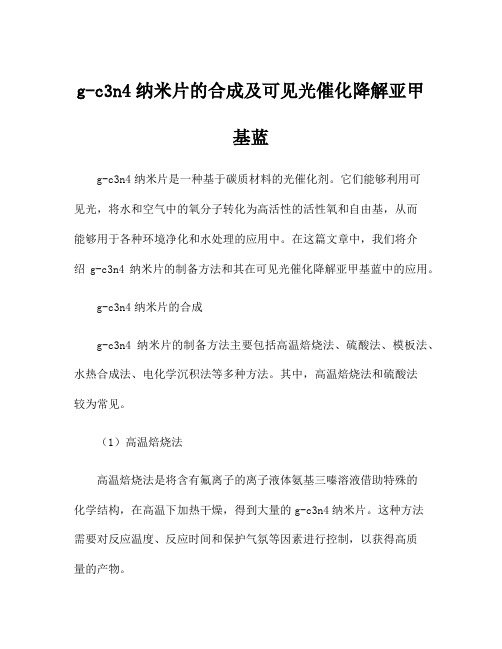
g-c3n4纳米片的合成及可见光催化降解亚甲基蓝g-c3n4纳米片是一种基于碳质材料的光催化剂。
它们能够利用可见光,将水和空气中的氧分子转化为高活性的活性氧和自由基,从而能够用于各种环境净化和水处理的应用中。
在这篇文章中,我们将介绍g-c3n4纳米片的制备方法和其在可见光催化降解亚甲基蓝中的应用。
g-c3n4纳米片的合成g-c3n4纳米片的制备方法主要包括高温焙烧法、硫酸法、模板法、水热合成法、电化学沉积法等多种方法。
其中,高温焙烧法和硫酸法较为常见。
(1)高温焙烧法高温焙烧法是将含有氟离子的离子液体氨基三嗪溶液借助特殊的化学结构,在高温下加热干燥,得到大量的g-c3n4纳米片。
这种方法需要对反应温度、反应时间和保护气氛等因素进行控制,以获得高质量的产物。
(2)硫酸法硫酸法则是在碱性环境中,将葡萄糖等有机小分子通过不断加热、干燥、转化而制备的。
该方法较简单,而且产出的g-c3n4纳米片在可见光下有较高的光催化活性。
g-c3n4纳米片的光催化应用g-c3n4纳米片是一种优秀的可见光催化剂,在环境净化、水处理和气体清洁等领域均有广泛的应用。
此处我们以可见光催化降解亚甲基蓝为例。
亚甲基蓝是一种常见的工业染料,具有毒性和致癌性,对人类和环境都有危害。
而g-c3n4纳米片可以利用可见光催化活性,在光照下促进亚甲基蓝的降解,从而实现其净化的目的。
在研究中,利用硫酸法制备了g-c3n4纳米片,并通过紫外-可见吸收光谱、X射线粉末衍射分析和透射电子显微镜等方法对产物进行了鉴定和表征。
随后,利用可见光催化实验测试了g-c3n4纳米片在降解亚甲基蓝中的性能。
结果显示,g-c3n4纳米片对亚甲基蓝的降解率可以高达90%,同时还具有较强的反应稳定性和重复使用性。
该研究揭示了g-c3n4纳米片在可见光催化领域的应用前景,并为相关工业及环保方面提供了重要的参考信息。
综上所述,g-c3n4纳米片是一种具有极高应用价值的材料。
g-c3n4基核壳结构复合光催化剂制备、表征及活性评价研究
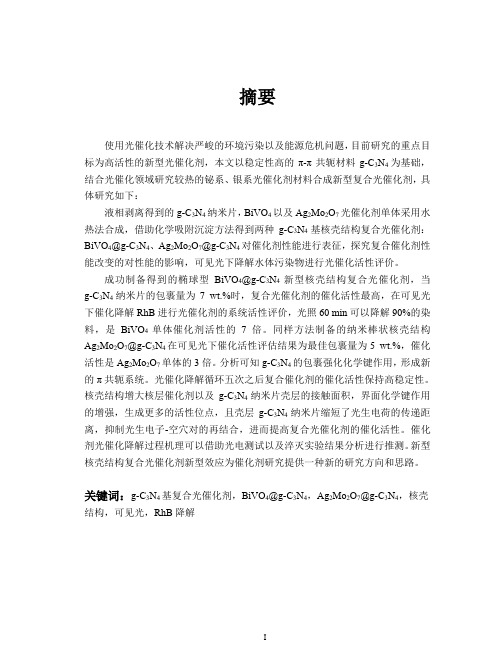
摘要使用光催化技术解决严峻的环境污染以及能源危机问题,目前研究的重点目标为高活性的新型光催化剂,本文以稳定性高的π-π共轭材料g-C3N4为基础,结合光催化领域研究较热的铋系、银系光催化剂材料合成新型复合光催化剂,具体研究如下:液相剥离得到的g-C3N4纳米片,BiVO4以及Ag2Mo2O7光催化剂单体采用水热法合成,借助化学吸附沉淀方法得到两种g-C3N4基核壳结构复合光催化剂:BiVO4@g-C3N4、Ag2Mo2O7@g-C3N4对催化剂性能进行表征,探究复合催化剂性能改变的对性能的影响,可见光下降解水体污染物进行光催化活性评价。
成功制备得到的椭球型BiVO4@g-C3N4新型核壳结构复合光催化剂,当g-C3N4纳米片的包裹量为7 wt.%时,复合光催化剂的催化活性最高,在可见光下催化降解RhB进行光催化剂的系统活性评价,光照60 min可以降解90%的染料,是BiVO4单体催化剂活性的7倍。
同样方法制备的纳米棒状核壳结构Ag2Mo2O7@g-C3N4在可见光下催化活性评估结果为最佳包裹量为5 wt.%,催化活性是Ag2Mo2O7单体的3倍。
分析可知g-C3N4的包裹强化化学键作用,形成新的π共轭系统。
光催化降解循环五次之后复合催化剂的催化活性保持高稳定性。
核壳结构增大核层催化剂以及g-C3N4纳米片壳层的接触面积,界面化学键作用的增强,生成更多的活性位点,且壳层g-C3N4纳米片缩短了光生电荷的传递距离,抑制光生电子-空穴对的再结合,进而提高复合光催化剂的催化活性。
催化剂光催化降解过程机理可以借助光电测试以及淬灭实验结果分析进行推测。
新型核壳结构复合光催化剂新型效应为催化剂研究提供一种新的研究方向和思路。
关键词:g-C3N4基复合光催化剂,BiVO4@g-C3N4,Ag2Mo2O7@g-C3N4,核壳结构,可见光,RhB降解IABSTRACTWith the challenge of environment pollution and energy crisis, photocatalytic technology is the possible solution. The aim of the research focus on the the novel photocatalyst with high activity. This article is based on the π-π conjugated g-C3N4 material with high stablility, combined with the materials like bismuth-based, silver-based photocatalyst, synthesis the new composite photocatalyst, more details are as follows:The g-C3N4nanosheet was obtained by the liquid exfoliated, BiVO4and Ag2Mo2O7were prepared by the hydrothermal method. BiVO4@g-C3N4and Ag2Mo2O7@g-C3N4core-shell composite photocatalysts were synthesized by chemical adsorption. The new composites were examined with various characterization techniques, the effects of the structure change on the performance of the photocatalyst were investigated. The photocatalytic activity assess was evaluated by degradating the water pollution under visible light.Novel core-shell structured ellipsoid-like BiVO4@g-C3N4 composites, its performance as photocatalyst was systematically evaluated during RhB degradation under visible light irradiation. The composite with 7 wt.% g-C3N4 was found to degradate almost 90% RhB in 60 min, which is 7 times more efficient as a photocatalyst than pristine BiVO4. As for nanorod Ag2Mo2O7@g-C3N4, when the amout of g-C3N4nanosheets reached 5 wt.%, Ag2Mo2O7@g-C3N4(5 wt.%) showed the highest degradation efficiency, which is 3 times more efficient than pristine BiVO4. The results of FTIR show core-shell structure modified chemical bands interactions, forming a wider πconjugated system. Their core-shell structure and activity were also found to be highly stable after it was used for 5 times in RhB degradation. The core-shell structure enhanced the contact areas between the BiVO4 core and g-C3N4 nanosheets shell, which provided more active sites and strengthened the chemical band interaction. The thin g-C3N4 nanosheets reduced the charge carrier transfer distance, which further suppressed the recombination of the photo-induced electron-hole pairs and therefore the photocatalytic activity of the composites enhanced. A reaction mechanism of the photocatalytic RhB degradation was proposed and discussed in detail. Not only the g-C3N4nanosheets coating improved theIIphotocatalytic activity, but also strengthened the stability.This promising novel core-shell composite photocatalyst provides an exciting area for further study and application.KEY WORDS:G-C3N4 based composite photocatalyst, BiVO4@g-C3N4,Ag2Mo2O7@g-C3N4, Core-shell structure, Visible light, RhB degradationIII目录第1章文献综述 (1)1.1 半导体光催化剂机理与应用 (1)1.1.1 半导体材料的带隙结构 (1)1.1.2 半导体光催化过程的机理 (2)1.1.3 半导体光催化剂性能的影响因素 (3)1.2 半导体光催化剂的制备与改性方法 (5)1.2.1 光催化剂的合成方法 (5)1.2.2 光催化剂的改性方法 (6)1.3g-C3N4基光催化剂 (7)1.4 新型半导体光催化剂的研究 (9)1.4.1 新型TiO2材料的合成 (9)1.4.2 氧化物和硫化物 (9)1.4.3 钒/钼/钨酸盐复合光催化剂材料 (9)1.5 课题研究内容 (10)1.5.1 研究目标 (10)1.5.2 研究内容 (11)第2章实验部分 (13)2.1 实验药品及仪器 (13)2.2 催化剂表征测试方法及仪器 (14)2.2.1X射线衍射 (14)2.2.2 场发射扫描电镜及能谱图 (14)2.2.3 透射电镜及选区电子衍射 (14)2.2.4 傅里叶红外吸收光谱 (15)2.2.5X射线电子能谱图 (15)2.2.6 紫外可见吸收光谱图谱 (15)2.2.7 荧光吸收光谱 (16)2.2.8 瞬时光电流响应 (16)2.2.9 电化学阻抗 (16)2.3 催化剂制备实验部分 (17)2.3.1g-C3N4及g-C3N4纳米片的合成 (17)IV2.3.2 钒酸铋的制备 (17)2.3.3 核壳结构复合光催化剂BiVO4@g-C3N4的合成 (17)2.3.4 钼酸银的制备 (18)2.3.5 核壳结构复合光催化剂Ag2Mo2O7@g-C3N4的合成 (18)2.4 催化剂活性评价实验方案 (19)第3章BiVO4@g-C3N4核壳结构复合催化剂表征及活性评价 (21)3.1BiVO4@g-C3N4催化剂材料表征 (21)3.1.1 样品XRD分析 (21)3.1.2SEM分析 (22)3.1.3TEM形貌分析 (23)3.1.4FT-IR图谱分析 (24)3.1.5UV-vis光谱图分析 (25)3.1.6XPS分析 (28)3.2 光催化活性实验结果分析 (30)3.2.1 复合光催化剂样品的催化活性评价 (30)3.2.2 催化剂的淬灭实验 (32)3.2.3 催化剂的稳定性实验 (33)3.3 催化剂光电特性测试 (34)3.3.1 荧光光谱分析 (34)3.3.2 光电流测试分析 (35)3.3.3 阻抗测试分析 (35)3.4 复合光催化剂BiVO4@g-C3N4降解机理分析 (36)3.5 本章小结 (38)第4章Ag2Mo2O7@g-C3N4核壳结构复合催化剂表征及活性评价 (39)4.1Ag2Mo2O7@g-C3N4催化剂材料表征 (39)4.1.1XRD分析 (39)4.1.2SEM形貌分析 (40)4.1.3TEM图分析 (41)4.1.4FT-IR光谱分析 (43)4.1.5UV-vis光谱图分析 (44)4.1.6XPS分析 (45)4.2 光催化剂活性评价分析 (47)4.2.1 复合光催化剂的催化活性评价 (47)4.2.2 催化剂淬灭实验 (50)V4.2.3 催化剂活性寿命实验 (51)4.3 光催化剂的光电性能测试 (52)4.3.1 荧光光谱分析 (52)4.3.2 光电流响应测试 (53)4.3.3 阻抗测试分析 (54)4.4 复合催化剂光催化降解机理分析 (54)4.5 本章小结 (56)第5章结论与展望 (57)5.1 研究结论 (57)5.2 课题展望 (58)参考文献 (59)发表论文和参加科研情况说明 (69)致谢 (71)VI第1章文献综述第1章文献综述当今时代处在蓬勃发展的阶段,工业的膨胀发展导致了一系列失衡问题的出现,目前能源短缺是全人类面临的一项重大挑战,同时科技进步引发的环境污染问题也亟待解决。
熔盐辅助法制备g-C_(3)N_(4)纳米结构及其光催化制氢性能
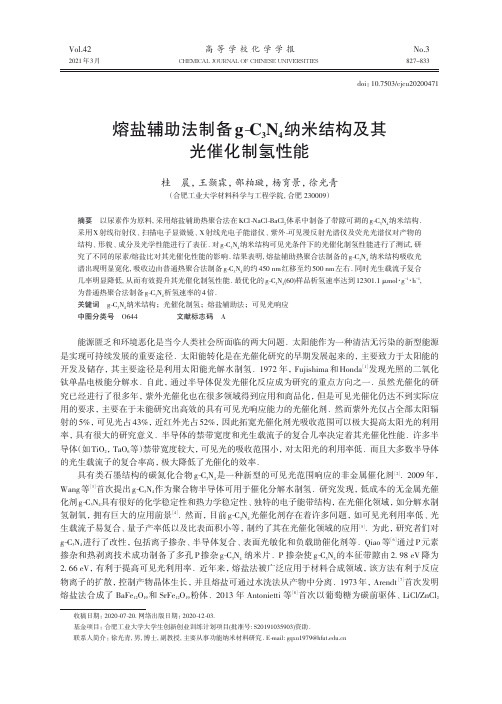
Vol.42 2021年3月No.3827~833 CHEMICAL JOURNAL OF CHINESE UNIVERSITIES高等学校化学学报熔盐辅助法制备g-C3N4纳米结构及其光催化制氢性能桂晨,王颢霖,邵柏璇,杨育景,徐光青(合肥工业大学材料科学与工程学院,合肥230009)摘要以尿素作为原料,采用熔盐辅助热聚合法在KCl-NaCl-BaCl2体系中制备了带隙可调的g-C3N4纳米结构.采用X射线衍射仪、扫描电子显微镜、X射线光电子能谱仪、紫外-可见漫反射光谱仪及荧光光谱仪对产物的结构、形貌、成分及光学性能进行了表征.对g-C3N4纳米结构可见光条件下的光催化制氢性能进行了测试,研究了不同的尿素/熔盐比对其光催化性能的影响.结果表明,熔盐辅助热聚合法制备的g-C3N4纳米结构吸收光谱出现明显宽化,吸收边由普通热聚合法制备g-C3N4的约450nm红移至约500nm左右.同时光生载流子复合几率明显降低,从而有效提升其光催化制氢性能.最优化的g-C3N4(60)样品析氢速率达到12301.1µmol∙g‒1∙h‒1,为普通热聚合法制备g-C3N4析氢速率的4倍.关键词g-C3N4纳米结构;光催化制氢;熔盐辅助法;可见光响应中图分类号O644文献标志码A能源匮乏和环境恶化是当今人类社会所面临的两大问题.太阳能作为一种清洁无污染的新型能源是实现可持续发展的重要途径.太阳能转化是在光催化研究的早期发展起来的,主要致力于太阳能的开发及储存,其主要途径是利用太阳能光解水制氢.1972年,Fujishima和Honda[1]发现光照的二氧化钛单晶电极能分解水.自此,通过半导体促发光催化反应成为研究的重点方向之一.虽然光催化的研究已经进行了很多年,紫外光催化也在很多领域得到应用和商品化,但是可见光催化仍达不到实际应用的要求,主要在于未能研究出高效的具有可见光响应能力的光催化剂.然而紫外光仅占全部太阳辐射的5%,可见光占43%,近红外光占52%,因此拓宽光催化剂光吸收范围可以极大提高太阳光的利用率,具有很大的研究意义.半导体的禁带宽度和光生载流子的复合几率决定着其光催化性能.许多半导体(如TiO2,TaO6等)禁带宽度较大,可见光的吸收范围小,对太阳光的利用率低.而且大多数半导体的光生载流子的复合率高,极大降低了光催化的效率.具有类石墨结构的碳氮化合物g-C3N4是一种新型的可见光范围响应的非金属催化剂[2].2009年,Wang等[3]首次提出g-C3N4作为聚合物半导体可用于催化分解水制氢.研究发现,低成本的无金属光催化剂g-C3N4具有很好的化学稳定性和热力学稳定性、独特的电子能带结构,在光催化领域,如分解水制氢制氧,拥有巨大的应用前景[4].然而,目前g-C3N4光催化剂存在着许多问题,如可见光利用率低、光生载流子易复合、量子产率低以及比表面积小等,制约了其在光催化领域的应用[5].为此,研究者们对g-C3N4进行了改性,包括离子掺杂、半导体复合、表面光敏化和负载助催化剂等.Qiao等[6]通过P元素掺杂和热剥离技术成功制备了多孔P掺杂g-C3N4纳米片.P掺杂使g-C3N4的本征带隙由2.98eV降为2.66eV,有利于提高可见光利用率.近年来,熔盐法被广泛应用于材料合成领域,该方法有利于反应物离子的扩散,控制产物晶体生长,并且熔盐可通过水洗法从产物中分离.1973年,Arendt[7]首次发明熔盐法合成了BaFe12O19和SrFe12O19粉体.2013年Antonietti等[8]首次以葡萄糖为碳前驱体、LiCl/ZnCl2doi:10.7503/cjcu20200471收稿日期:2020-07-20.网络出版日期:2020-12-03.基金项目:合肥工业大学大学生创新创业训练计划项目(批准号:S20191035903)资助.联系人简介:徐光青,男,博士,副教授,主要从事功能纳米材料研究.E-mail:*****************.cn828Vol.42高等学校化学学报为熔盐,经过裂解得到纳米孔炭材料.2017年,曲晓钰等[9]利用熔盐法进行镍掺杂的石墨相氮化碳合成研究,得到了较大比表面积并在可见光下具有较高光催化固氮性能的镍离子掺杂石墨相氮化碳催化剂.本文以尿素为前驱体,在KCl-NaCl-BaCl2三元熔盐体系中合成了具有良好可见光响应的g-C3N4纳米片,并对其可见光催化析氢性能进行了研究.1实验部分1.1试剂尿素、氯化钠、氯化钾、氯化钡、无水乙醇、氯铂酸和三乙醇胺均购自阿拉丁试剂(上海)有限公司,所有试剂均为分析纯;实验用水均为自制去离子水.1.2样品的制备将50g尿素和一定量的混合盐(NaCl/KCl/BaCl质量比为2∶3∶5)溶于200mL去离子水中,并进行搅拌,超声处理直至完全溶解,获得混合液.使用DC-1500实验型喷雾干燥机将混合溶液喷雾造粒,形成尿素和盐的前驱体混合物.将一定量前驱体混合物装入氧化铝瓷舟中,并置于箱式炉中,以7℃/min的升率速度升温至550℃,保温2h后随炉冷却.冷却至室温后收集产物,并分散于去离子水中,充分溶解其中的混合盐.经离心去除上层清液,反复清洗7次后收集固体并置于60℃烘箱中干燥12h,制得所需的产物.按照熔盐在混合前驱体所占质量分数(0,10%,40%,60%和80%),将制得的产物分别命名为g-C3N4,g-C3N4(10),g-C3N4(40),g-C3N4(60)和g-C3N4(80)纳米片.1.3样品的表征采用D/MAX2500V型X射线衍射仪(荷兰帕纳科公司)分析样品的物相组成及结晶情况,扫描范围为5°~90°,扫描速率为2°/min;通过SU8020型冷场发射扫描电子显微镜(日本日立公司)观察样品表面的微观形貌,测试前将样品分散在导电胶基体上,并表面喷金30s;采用ESCALAB250Xi型X射线光电子能谱仪(美国Thermo公司)分析产物的表面成分和元素的价态;采用UV-3600型紫外-可见漫反射光谱仪(日本岛津公司)测试产物的光吸收性能,并估算样品禁带宽度,扫描范围为200~900nm;采用F-4500型荧光光谱仪(日本日立公司)测试样品的光致发光谱,以分析产物的光生载流子的复合几率,激发波长为340nm;采用NONA4200e型气体吸附仪(美国康塔公司)分析不同样品的比表面积.1.4光解水制氢性能测试采用北京泊菲莱公司的Labsolar-III AI型光催化系统作为样品的光解水制氢反应装置,并保持整个反应过程中玻璃系统处于真空状态.将10mg待测样品分散于装有90mL去离子水的石英玻璃反应器中,加入10mL的三乙醇胺作为空穴捕获剂,以Pt作为助催化剂,按照Pt的质量分数为3%加入153µL 的氯铂酸.检测反应系统气密性,开启真空泵对反应系统抽真空,然后密闭反应系统.用装有400nm 截止滤波片的300W氙灯作为光源,高纯氩气为载气.反应过程中,通过磁力搅拌使反应器中物质充分混合均匀,并在冷却循环水辅助下进行整个反应过程,反应产物通过FL-9790型气相色谱仪(浙江福立分析仪器股份有限公司)收集、分析.所有样品的光催化析氢测试进行2h,每30min采集析氢信号,分析制氢速率.2结果与讨论2.1样品的形貌表征图1所示为不同熔盐加入量所制备产物的X射线衍射(XRD)谱图.由图1可见,直接煅烧产物(g-C3N4)的XRD谱图在2θ=12.7°和27.5°处存在2个特征峰,分别对应于典型的面内三嗪环重复结构的(100)晶面和共轭芳香体系的层间堆垛的(002)晶面[4],表明通过以尿素为前驱体的热聚合反应成功制备出了类石墨结构g-C3N4.而采用熔盐辅助法所制产物的XRD谱图发生较大变化,4个样品中均未出No.3桂晨等:熔盐辅助法制备g -C 3N 4纳米结构及其光催化制氢性能现(100)衍射峰,其(002)衍射峰强度也明显降低,表明反应过程中的熔盐辅助作用导致了层间堆垛的有序程度降低,纳米片更薄.随着混合前驱体中熔盐质量分数的增加,样品中出现了明显的新衍射峰,其与BaCO 3的标准卡片(PDF No.41-0373)一致,表明含盐量高时煅烧过程中生成了BaCO 3,最终残留在样品中.采用扫描电子显微镜对直接煅烧制备的g -C 3N 4和熔盐辅助制备的g -C 3N 4(10),g -C 3N 4(40)和g -C 3N 4(60)样品进行了形貌观察,结果示于图2中.直接煅烧的g -C 3N 4具有松散的二维类石墨结构,实测比表面积(64.5m 2/g )较高,其形貌及表面积均与文献[10]报道结果基本一致.经熔盐辅助法制备的样品,分散性较差,在相同质量情况下其体积明显小于直接煅烧的g -C 3N 4,并存在较为严重的团聚现象.实测g -C 3N 4(60)样品比表面积有所减少,仅为36.0m 2/g ,表明熔盐辅助过程中产物的分散性、比表面积有一定程度的降低.图3所示为直接煅烧g -C 3N 4和g -C 3N 4(60)样品的X 射线光电子能谱图(XPS ).其中图3(A )为g -C 3N 4和g -C 3N 4(60)的XPS 全谱,显示g -C 3N 4样品中主要存在C ,N 和O 3种元素,而g -C 3N 4(60)样品中除了C ,N 和O 元素之外,还存在Na ,K 和Ba 等金属元素.图3(B )~(D )分别为g -C 3N 4和g -C 3N 4(60)中C 1s ,N 1s 和O 1s 电子的XPS 高分辨谱图,对其进行了高斯分解,结果示于表1中.由图3(B )中C 1s 的高分辨XPS 谱图分析可知,直接煅烧制备g -C 3N 4纳米片中的C 1s XPS 谱可以分解为288.0,286.5和284.8eV 3个峰,可分别对应于芳香结构的N―C =N 键、C―NH键和游离C―C 键[11~13],其中游离的C―C 键来源于测试过程.3种结构中C 所占的比例示于表1中,属于g -C 3N 4结构中的C 占比为90.5%.g -C 3N 4(60)中C 1s 的XPS 谱中比g -C 3N 4纳米片的少了一个位于286.5eV 处的结合能峰,表明该样品中不存在C―NH 结构;同时多出了一个位于289.5eV 的峰,该结合能峰源于C―O 结合中的C [14,15],结合XRD 结果可知应为BaCO 3中的C.同时各个C 峰的相对强度发生了变化,属于g -C 3N 4基本结构的C 占比仅为57.5%.图3(C )所示为2种样品中N 1s 的高分辨XPS 谱图.g -C 3N 4中的N 可以分解成结合能位于398.5,399.8和401.1eV 的3个峰,分别对应于C―N =C 结构、N —(C )3结构和末端氨基N―H 结构中的N [11,12].g -C 3N 4(60)样品中N 1s 的XPS 谱图由400.1和398.4eV 2个峰组成,均来自于g -C 3N 4中基本结构单元中的C―N =C 结构和N —(C )3结构,401.1eV 结合能峰的消失意味着在g -C 3N 4(60)样品中基本不存在末端氨基N―H 结构.2种样品的O 1s 电子结合能位置出现了明显变化,如图3(D )所示,经分解后均可获得位于532.8和531.3eV 的2个峰,其中前者属于N―OH 结构,后者属于C =O 结构.直接煅烧g -C 3N 4纳米片中O 以532.8eV 峰为主,主要来源于纳米片的表面羟基,熔盐辅助制备的g -C 3N 4(60)样品中O 以531.3eV峰Fig.2SEM images of direct⁃calcination g⁃C 3N 4(A)and g⁃C 3N 4(10)(B),g⁃C 3N 4(40)(C)and g⁃C 3N 4(60)(D)samplesFig.1XRD patterns of direct⁃calcination g⁃C 3N 4(a ),g ⁃C 3N 4(10)(b ),g ⁃C 3N 4(40)(c ),g ⁃C 3N 4(60)(d ),g⁃C 3N 4(80)(e )and BaCO 3(f )829Vol.42高等学校化学学报为主,结合XRD 谱图以及C 的XPS 谱图可知,该O 元素主要来源于残留的BaCO 3.通过XPS 测试获得各元素的相对含量示于表2中,考虑到C 元素包括测试过程引入的C 以及BaCO 3中的碳,按照表1中各种C 所占的比例,计算出属于C 3N 4基本结构中的C 含量(见表中括号内数据).由此获得直接煅烧g -C 3N 4纳米片的C/N 摩尔比为0.74,基本与标准C 3N 4的成分相吻合.g -C 3N 4(60)纳米片的C/N 摩尔比为0.81,略高于标准的0.75,主要原因是熔盐辅助过程中的脱氨基行为降低了N 含量.2.2样品的光催化析氢性能以三乙醇胺作为牺牲剂在可见光(λ>400nm )下对样品光催化析氢性能进行测试,结果示于图4中.图4(A )所示为不同样品在可见光照射不同时间的产氢量,重复实验给出了不同样品产氢量的误差Fig.3XPS patterns of direct⁃calcination g⁃C 3N 4and g⁃C 3N 4(60)samples(A)XPS survey spectrum;high⁃resolution spectra of C 1s (B),N 1s (C)and O 1s (D).Table 1Gaussian decomposition results of C 1s ,N 1s and O 1s electrons in direct -calcination g -C 3N 4and molten -salt -assistance g -C 3N 4(60)samplesElement C 1sN 1s O 1sGroup C―ON―C =N C―NH C―C ―NH x N ―(C )3C―N =C N―OC =O g⁃C 3N 4E b /eV ―288.0286.5284.8401.0399.8398.5532.8531.3Molar fraction (%)―83.86.79.56.523.769.872.727.3g⁃C 3N 4(60)E b /eV 289.7287.9―284.8―400.1398.4532.8531.3Molar fraction (%)4.657.5―37.9―18.181.915.484.6Table 2C,N,O contents and C/N molar ratios in the basic structure of g -C 3N 4and g -C 3N 4(60)specimensSample g⁃C 3N 4g⁃C 3N 4(60)Elemental content (%)C42.88(38.8)42.91(24.6)N52.2130.57O4.9118.91C/N molar ratio0.740.81830No.3桂晨等:熔盐辅助法制备g -C 3N 4纳米结构及其光催化制氢性能棒.其中g -C 3N 4(60)样品经2h 光照后的析氢总量达到24116µmol ,远远高于直接煅烧g -C 3N 4的析氢总量.图4(B )所示为g -C 3N 4和不同熔盐比例制备产物的平均析氢速率,其中直接煅烧的g -C 3N 4纳米片析氢速率为2789.4µmol∙g ‒1∙h ‒1,析氢速率明显高于采用三聚氰胺为原料直接煅烧制得的g -C 3N 4,主要原因在于尿素为原料直接煅烧产物比表面积相对较高[16].熔盐辅助制备的g -C 3N 4样品析氢性能均明显高于直接煅烧样品,如g -C 3N 4(10)样品的平均析氢速率为4416.5µmol∙g ‒1∙h ‒1,并且随着熔盐比例的增加,析氢速率明显增加.当熔盐质量分数为40%时,样品平均析氢速率为6683.8µmol∙g ‒1∙h ‒1,尤其是熔盐质量分数达到60%时,所制备的g -C 3N 4(60)样品具有最高的析氢速率12301.1µmol∙g ‒1∙h ‒1,为直接煅烧g -C 3N 4纳米片析氢速率的4倍左右,性能增加明显.进一步提高熔盐质量分数到80%,样品析氢速率降至6335.2µmol∙g ‒1∙h ‒1.同时,因为原料占比较少,产物量较少,难以收集.上述析氢性能测试表明,通过优化熔盐辅助工艺可以实现g -C 3N 4析氢性能的明显提升,而且从宏观样品来看其颜色由直接煅烧产物的淡黄色变成橙黄色,而样品的光吸收性能是影响光催化性能的一个重要因素.对不同熔盐成分制备的g -C 3N 4样品进行紫外-可见光区域的光吸收性能及光致发光性能进行测试,结果示于图5中.图5(A )所示为不同样品的紫外-可见吸收光谱,直接煅烧的g -C 3N 4纳米片具有良好的紫外光吸收性能,其吸收边位于466nm ,具有一定的可见光吸收性能,但总体只能吸收波长在蓝光以下的少量可见光.经过熔盐辅助制备的样品出现了明显的紫外吸收增强和吸收边红移的现象,红移波长为20nm 以上,这种吸收边的红移使得其可见光吸收性能明显提升.根据Kubelka -Munk 法进行吸收光谱的数据转化,获得(ahv )1/2-hv 曲线,如图5(B )所示.直接煅烧g -C 3N 4的带隙宽度为3.04eV ,熔盐辅助获得的样Fig.4Photocatalytic hydrogen evolution performance of direct⁃calcination g⁃C 3N 4and g⁃C 3N 4(10),g⁃C 3N 4(40),g⁃C 3N 4(60)and g⁃C 3N 4(80)samples(A)H 2production;(B)hydrogen evolution rate.Fig.5UV⁃Vis absorption spectra(A),(ahv )1/2⁃hv curves(B)and photoluminescence patterns(C)of direct⁃calcination g⁃C 3N 4and g⁃C 3N 4(10),g⁃C 3N 4(40),g⁃C 3N 4(60)and g⁃C 3N 4(80)samples(B)Band gap/eV:g⁃C 3N 4:3.04;g⁃C 3N 4(10):2.72;g⁃C 3N 4(40):2.77;g⁃C 3N 4(60):2.80;g⁃C 3N 4(80):2.80.831832Vol.42高等学校化学学报品禁带宽度均有明显降低,位于2.7~2.8eV之间,表明熔盐辅助过程可以缩小g-C3N4的带隙宽度.图5(C)所示为不同g-C3N4的光致发光光谱,用以反应光催化过程中在320nm紫外光激发下载流子的复合几率,低的发光强度意味着低的载流子复合几率.由图可见,直接煅烧g-C3N4纳米片具有较高的光致发光强度,即光生电子-空穴对复合几率较高,从而光催化析氢效率较低.熔盐辅助制备的g-C3N4样品均具有较低的光致发光强度,表明其载流子复合几率很低,大大提高了其光催化制氢效率.碱金属离子掺杂在氮化碳材料层间形成插层,产生附加的键合作用,从而缩短层间距离,相邻层的紧密堆积有利于提高光捕获和加速光生载流子层间传递,使吸收边红移,抑制电子与空穴的复合. Xiong等[17]的研究表明,K原子和Na原子不参与带边结构的形成,但均可以有效扩大可见光吸收范围、缩小禁带宽度.Na和K原子在费米能级附近贡献了额外的电子,从而缩小带隙.通过对熔盐比例的调节,可有效控制导带与价带的位置,促进H2气的产生.g-C3N4在层间与层内具有大的静电势,阻止了电荷转移.掺杂Na和K后,g-C3N4的势垒明显降低,电荷载流子在层间、层内的传输更加容易[8].同时,K插层到g-C3N4层内,与相邻两层原子发生化学结合,形成电荷运输通道,从而降低电子局部化程度[17].在可见光激发下,g-C3N4样品内部的光激发电子-空穴对通过K沿层间方向迅速转移,使电子和空穴做定向迁移[18],促进电荷载流子的转移和分离,阻碍电子与空穴的复合.综上所述,采用熔盐辅助制备的g-C3N4纳米片具有更好的可见光吸收能力和高的载流子分离效率,从而具有更高的光催化析氢性能.有研究表明,K离子掺杂可以显著提高g-C3N4样品比表面积[19],但实验中实测g-C3N4(60)的比表面积较低,主要是因为样品中存在大量的残留BaCO3所致.此外BaCO3的存在可能会覆盖催化剂表面的活性位点,从而影响析氢性能[20].3结论采用熔盐辅助热聚合法成功制备了带隙可调的g-C3N4纳米片,并对其光催化析氢性能进行了研究,主要结论如下:采用熔盐辅助制备的g-C3N4纳米片基本保持与直接煅烧产物相同的结构,但层间堆垛有序性降低,并且样品中低的氨基含量也表明其类石墨层状结构中层厚较薄;与直接煅烧样品相比,熔盐辅助制备的g-C3N4纳米片均具有更高的可见光催化析氢性能,其中最优化的g-C3N4(60)纳米片析氢效率为12301.1µmol∙g‒1∙h‒1,为直接煅烧样品的4倍多;良好的可见光吸收能力和高的载流子分离效率是可见光催化析氢性能提升的关键因素.参考文献[1]Fujishima A.,Honda K.,Nature,1972,238(5358),37—38[2]Liang R.Y.,Xu D.D.,Zha W.Y.,Qi J.Z.,Huang L.H.,Chem.J.Chinese Universities,2016,37(11),1953—1959(梁瑞钰,徐冬冬,查文莹,齐楫真,黄浪欢.高等学校化学学报,2016,37(11),1953—1959)[3]Wang X.,Maeda K.,Thomas A.,Takanabe K.,Xin G.,Carlsson Johan M.,Domen K.,Antonietti M.,Nat.Mater.,2009,8(1),76—80[4]Zhao Y.F.,Sun X.L.,Hu S.Z.,Wang H.,Wang F.,Li P.,Chem.J.Chinese Universities,2020,41(1),132-139(赵艳锋,孙效龙,胡绍争,王辉,王菲,李萍.高等学校化学学报,2020,41(1),132—139)[5]Jiang S.K.,Meng X.Z.,Agriculture and Technology,2020,40(13),26—28(姜淑坤,孟祥哲.农业与技术,2020,40(13),26—28)[6]Ran J.R.,Ma T.Y.,Gao G.P.,Duc X.W.,Qiao S.Z.,Energy Environ.Sci.,2015,8(12),3708—3717[7]Arendt R.H.,J.Solid State Chem.,1973,(8),339—347[8]Fechler N.,Wohlgemuth S.A.,Jäker P.,Antonietti M.,J.Mater.Chem.A,2013,1(33),9418—9421[9]Qu X.Y.,Hu S.Z.,Li P.,Wang F.,Zhao Y.F.,Wang Q.,Chem.J.Chinese Universities,2017,38(12),2280—2288(曲晓钰,胡绍争,李萍,王菲,赵艳锋,王琼.高等学校化学学报,2017,38(12),2280—2288)[10]Liu Z.M.,Zhao C.C.,Wang S.J.,Guo R.,Wang Y.J.,Han J.P.,Acta Petrolei Sinica(Petroleum Processing Section),2018,34(2),365—372(刘宗梅,赵朝成,王帅军,郭锐,王永剑,韩建鹏.石油学报(石油加工),2018,34(2),365—372)[11]Yang Y.,Wang S.,Jiao Y.,Wang Z.,Xiao M.,Du A.,Li Y.,Wang J.,Wang L.,Adv.Funct.Mater.,2018,28(47),1805698[12]Ruan D.,Kim S.,Fujitsuka M.,Majima T.,Appl.Catal.B:Environ.,2018,238(15),638—646[13]Jiang Y.,Sun Z.,Tang C.,Zhou Y.,Zeng L.,Huang L.,Appl.Catal.B:Environ.,2019,240,30—38[14]Wang Y.,Li Y.,Zhao J.,Wang J.,Li Z.,Int.J.Hydrogen Energ.,2019,44(2),618—628[15]Liu J.,Fang W.,Wei Z.,Qin Z.,Jiang Z.,Shangguan W.,Appl.Catal.B:Environ.,2018,238(15),465—470[16]Fan C.,Feng Q.,Xu G.,Lv J.,Zhang Y.,Liu J.,Qin Y.,Wu Y.,Appl.Surf.Sci.,2018,427,730—738No.3桂晨等:熔盐辅助法制备g -C 3N 4纳米结构及其光催化制氢性能[17]Xiong T.,Cen W.,Zhang Y.,Dong F.,ACS Catal.,2016,6(4),2462—2472[18]Wu M.,Zhang J.,He B.B.,Wang H.W.,Wang R.,Gong Y.S.,Appl.Catal.B :Environ.,2019,241,159—166[19]Wang H.,Pei Y.B.,Hu S.Z.,Ma W.T.,Shi S.Y.,Chem.J.Chinese Universities ,2018,39(7),1503—1510(王辉,裴彦博,胡绍争,马文涛,石朔宇.高等学校化学学报,2018,39(7),1503—1510)[20]Zeng Z.,Yu H.,Quan X.,Chen S.,Zhang S.,Appl.Catal.B :Environ.,2018,227(5),153—160Molten -salt -assistance Synthesis and Photocatalytic HydrogenEvolution Performances of g -C 3N 4Nanostructures †GUI Chen ,WANG Haolin ,SHAO Baixuan ,YANG Yujing ,XU Guangqing *(School of Materials Science and Engineering ,Hefei University of Technology ,Hefei 230009,China )AbstractUsing urea as raw material ,g -C 3N 4nanostructures with adjustable bandgap were prepared inKCl -NaCl -BaCl 2system by molten -salt -assistance thermal polymerization method.The structure ,morphology ,composition and optical properties of the products were characterized by X -ray diffraction ,scanning electron microscope ,X -ray photoelectron spectrometer ,UV -Visible diffuse reflection spectrometer and fluorescence spectrometer ,respectively.The photocatalytic performance of the products in visible light was tested ,and the effects of different urea/molten salt ratios on the photocatalytic performance of g -C 3N 4nanostructures were studied.The results show that the absorption spectra of g -C 3N 4nanostructure prepared by molten -salt -assistance thermal polymerization manifest obvious broadening ,and the absorption edge shifts from ca .450nm to ca .500nm ,compared with the g -C 3N 4prepared by ordinary thermal polymerization method.At the same time ,the recombination rate of photogenerated carriers is obviously reduced ,so the photocatalytic hydrogen production performance is effectively improved.The hydrogen evolution rate of the optimized g -C 3N 4(60)sam⁃ples reach 12301.1µmol∙g ‒1∙h ‒1,which is 4times that of g -C 3N 4prepared by ordinary thermal polymerization method.Keywordsg -C 3N 4nanostructures ;Photocatalytic hydrogen evolution ;Molten -salt -assistance method ;Visible light response (Ed.:V,K,S)Innovation and Entrepreneurship Training Program Project of Hefei University of Technology ,China833。
- 1、下载文档前请自行甄别文档内容的完整性,平台不提供额外的编辑、内容补充、找答案等附加服务。
- 2、"仅部分预览"的文档,不可在线预览部分如存在完整性等问题,可反馈申请退款(可完整预览的文档不适用该条件!)。
- 3、如文档侵犯您的权益,请联系客服反馈,我们会尽快为您处理(人工客服工作时间:9:00-18:30)。
中图分类号: O 643.36
文献标志码: A
文章编号: 1672–6146(2018)03–0023–04
Preparation and characterization of graphite-like Carbon Nitride
Zhou Shuhui, Liu Shikai, Liu Xinxin (School of Materials Science and Engineering, Henan University of Technology, Zhengzhou 450001, China)
通信作者: 刘世凯, shikai_liu@。收稿日期: 2018–03–30 基金项目: 河南省教育厅自然科学项目(14B430019, 18B430005)。
24
湖南文理学院学报(自然科学版)
2018 年
1 试验
1.1 g-C3N4 的制备
分别称取 10 g 尿素于 80 ℃干燥 24 h, 取出后研磨, 再将盛有尿素的氧化铝坩埚置于电阻炉中煅烧, 分别研究反应温度、升温速度和保温时间对样品的影响,其中反应温度考察 450、500、530、560 ℃, 升 温速度考察 1、3、5℃/min, 保温时间考察 2、3、5 h, 反应结束后随炉自然冷却, 然后将所得样品称重, 计算产率, 观察外观性状, 在无水乙醇中充分超声分散 30 min, 离心, 干燥备用。
近年来, 半导体光电转换或光催化材料方面的研究成为了国内外关注的热点[1]。作为一种新型的半 导体光电材料, 石墨相氮化碳(g-C3N4)引起了人们的广泛关注[2]。常温下 g-C3N4 属于类石墨的层片状结 构, 具有无毒、稳定性好、可见光响应等众多优点[3–4], 被广泛应用于光催化剂、催化剂载体、传感器 等领域[5–7]。g-C3N4 的制备方法主要包括电化学沉积法、热聚合法、固相反应法、溶剂热法等[8–10]。祝 佳鑫等[11]以二氰二胺与尿素的复合粉末为前驱体, 通过高温烧结法制备了具有典型多孔结构的 g-C3N4, 相比于纯二氰二胺或纯尿素所制备的产物具有更高的比表面积; 胡玉洪等[12]用高温煅烧三聚氰胺的方 法制备了黄色粉末状的 g-C3N4, 此法操作方便并且 g-C3N4 产率较高; 张胜等[13]采用溶剂热法合成了高 比表面积的 g-C3N4, 且光电流响应值较高, 阻抗值较小, 电催化活性较强。在 g-C3N4 的制备方法中, 热 聚合法对实验设备要求较低, 过程简单易行, 产率高, 适于大规模工业生产, 逐渐成为制备 g-C3N4 的常 用方法[14]。本文采用热聚合法, 以尿素作为原料制备 g-C3N4, 主要研究反应温度、升温速度、保温时间 对产物的影响, 以得到合成 g-C3N4 的最佳条件和制备规律, 并对优化条件下制备的样品在模拟太阳光 环境中研究其光催化的性质。
1.2 样品表征
物相分析采用 D8 ADVANCEECO 型自动 X 射线衍射仪(德国 BRUKER 公司), 样品成分测定采用 IRPrestige-21 型红外光谱仪(FTIR)(日本岛津公司), 样品形貌特征的观察采用扫描电镜(日立 S4800)。
Abstract: By using urea as precursor, the optimum conditions of g-C3N4 is fabricated at different reaction temperature, heating rate and holding time to explore the optimal conditions. The result shows that the optimum conditions are listed as follows: reaction temperature 500 ℃, heating rate 3 ℃/min, holding time 2 h. The photocatalytic performance of g-C3N4 prepared under optimal conditions is carried out under simulated sunlight, with the prolongation of time, the degradation rate is gradually increased, and the degradation rate reaches 93.2% after 4 h of light irradiation, which has good photocatalytic properties. Key words: graphite-like Carbon Nitride; thermal polymerization; characterization; photocatalysis
第 30 卷第 3 期 2018 年 9 月
湖南文理学院学报(自然科学版) Journal of Hunan University of Arts and Science(Science and Technology)
doi: 10.3969/j.issn.1672–6146.2018.03.006
Vol. 30 No. 3 Sep. 2018来自g-C3N4 的制备及表征
周淑慧, 刘世凯, 刘鑫鑫
(河南工业大学 材料科学与工程学院, 河南 郑州, 450001)
摘要: 以尿素为前驱体, 以不同的反应温度、升温速度、保温时间制得 g-C3N4, 探索制备 g-C3N4 的最优条件。 结果表明: 制备 g-C3N4 的最优条件为反应温度 500 ℃、升温速度 3 ℃/min、保温时间 2 h; 将最优条件下制得 的 g-C3N4 在模拟太阳光环境中进行光催化测试, 随着时间的延长, 降解率逐渐提高; 光照 4 h 的降解率达 93.2%, 这表明 g-C3N4 具有良好的光催化特性。 关键词: g-C3N4; 热聚合; 表征; 光催化