A sensitive TLRH targeted imaging technique for ultrasonic molecular imaging
科技使人进步英语作文

科技使人进步英语作文Technology has always been a driving force behind human progress. The advancement of technology has not only transformed the way we live work and communicate but it has also opened up new horizons for learning innovation and exploration. Here is an essay that delves into the impact of technology on human progress.Title The Impact of Technology on Human ProgressIntroductionIn the modern era technology has become an integral part of our lives. From the moment we wake up to the time we go to sleep technology is there facilitating our daily activities and making our lives easier. The progress of technology has been a catalyst for change pushing humanity forward and opening up new possibilities that were once only imagined.The Role of Technology in EducationOne of the most significant impacts of technology on human progress is in the field of education. With the advent of the internet access to knowledge has become virtually limitless. Online learning platforms educational apps and digital libraries have made it possible for anyone anywhere to learn new skills and acquire knowledge at their own pace. This democratization of education has empowered individuals and communities breaking down barriers of distance and socioeconomic status.Innovation and Economic GrowthTechnology has also been a key driver of economic growth and innovation. The development of new technologies has created new industries and job opportunities while also making existing industries more efficient and competitive. For example the rise of automation and artificial intelligence has revolutionized manufacturing logistics and service sectors leading to increased productivity and cost savings.Healthcare AdvancementsIn healthcare technology has transformed the way diseases are diagnosed treated and managed. Medical imaging technologies advanced surgical tools and personalizedmedicine have improved patient outcomes and reduced the burden on healthcare systems. Telemedicine and remote monitoring have made healthcare more accessible particularly for those living in remote areas or with limited mobility.Environmental BenefitsEnvironmental sustainability is another area where technology has made significant strides. Renewable energy technologies such as solar panels and wind turbines have become more efficient and affordable reducing our reliance on fossil fuels and mitigating the impact of climate change. Additionally advancements in materials science and engineering have led to the development of ecofriendly products and processes that minimize waste and pollution.Challenges and ConsiderationsWhile the benefits of technology are vast it is important to acknowledge the challenges it presents. Issues such as privacy concerns digital divide and the potential for job displacement due to automation require thoughtful consideration and proactive solutions. It is crucial for policymakers educators and industry leaders to work together to ensure that the benefits of technology are equitably distributed and that its potential negative impacts are mitigated.ConclusionIn conclusion technology has been and continues to be a powerful force for human progress. It has the potential to improve our quality of life drive economic growth and address some of the worlds most pressing challenges. As we continue to innovate and develop new technologies it is essential that we do so with a focus on inclusivity sustainability and ethical considerations to ensure that the benefits of technological advancement are felt by all.This essay highlights the multifaceted impact of technology on various aspects of human life emphasizing the importance of responsible and thoughtful advancement in this everevolving field.。
智能芯片到脑子里去,英语作文
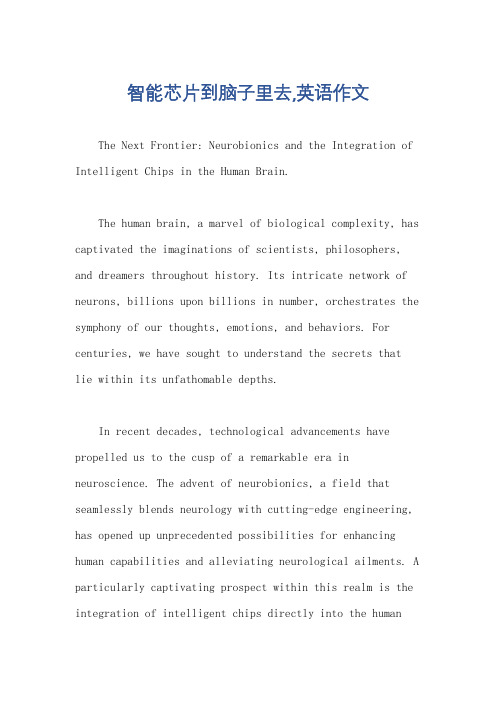
智能芯片到脑子里去,英语作文The Next Frontier: Neurobionics and the Integration of Intelligent Chips in the Human Brain.The human brain, a marvel of biological complexity, has captivated the imaginations of scientists, philosophers, and dreamers throughout history. Its intricate network of neurons, billions upon billions in number, orchestrates the symphony of our thoughts, emotions, and behaviors. For centuries, we have sought to understand the secrets thatlie within its unfathomable depths.In recent decades, technological advancements have propelled us to the cusp of a remarkable era in neuroscience. The advent of neurobionics, a field that seamlessly blends neurology with cutting-edge engineering, has opened up unprecedented possibilities for enhancing human capabilities and alleviating neurological ailments. A particularly captivating prospect within this realm is the integration of intelligent chips directly into the humanbrain.Envision a scenario where a minuscule, yet potent, microchip is implanted into the brain. This chip, equipped with sophisticated algorithms and advanced connectivity, would possess the remarkable ability to monitor neural activity in real-time, analyze patterns, and respond with targeted interventions. Such a device could revolutionize our approaches to a wide spectrum of neurological conditions.One such condition, epilepsy, characterized by recurrent seizures, affects millions worldwide. Current treatment modalities, often involving anticonvulsant medications, can be challenging to manage and may come with undesirable side effects. The integration of intelligent chips could provide a more effective and personalized approach. By monitoring brain activity continuously, the chip could detect the onset of seizures and deliver precisely timed electrical impulses or pharmacological interventions to prevent or mitigate them.Similarly, neurodegenerative diseases such as Alzheimer's and Parkinson's could potentially benefit from this technology. These debilitating conditions arise from the progressive loss of neurons, leading to cognitive impairment, movement disorders, and a decline in overall quality of life. Intelligent chips could be employed to compensate for neuronal loss by stimulating specific brain areas or intervening to slow down disease progression.The potential applications of intelligent chips in the human brain extend far beyond the realm of clinical medicine. As our understanding of neural circuits continues to expand, the possibility of augmenting human cognition and sensory perception becomes tantalizingly close. By enhancing neural processing and providing real-time feedback, chips could facilitate accelerated learning, improved memory function, and heightened sensory acuity.For instance, individuals with visual impairments could benefit from chips that amplify neural signals in thevisual cortex, enhancing their ability to perceive objects and navigate their surroundings. Similarly, chips implantedin the auditory cortex could restore hearing in those with hearing loss.The integration of intelligent chips into the human brain also presents a path towards a deeper understanding of ourselves. By providing a window into the intricate workings of the mind, chips could facilitate real-time analysis of neural activity, shedding light on the neural underpinnings of consciousness, decision-making, and emotional experiences.However, it is crucial to acknowledge that the pursuit of neurobionics comes with a myriad of ethical, social, and safety considerations that must be carefully weighed. The implantation of foreign devices into the human body raises concerns about potential risks and long-term complications. Ethical guidelines must be established to ensure that neurobionics is employed for the benefit of humanity, not to the detriment of individuals or society.As we navigate the uncharted waters of neurobionics, international collaboration and interdisciplinary researchwill be paramount. Scientists, engineers, ethicists, and policymakers must work hand-in-hand to establish clear frameworks for the responsible development and clinical application of intelligent chips in the human brain.The integration of intelligent chips into the human brain holds the promise of transformative advancements in healthcare, human enhancement, and our understanding of the human condition. By embracing a thoughtful and inclusive approach, we can harness the power of neurobionics to elevate human potential and pave the way for a brighter, more fulfilling future for all.。
病灶定位技术的英语
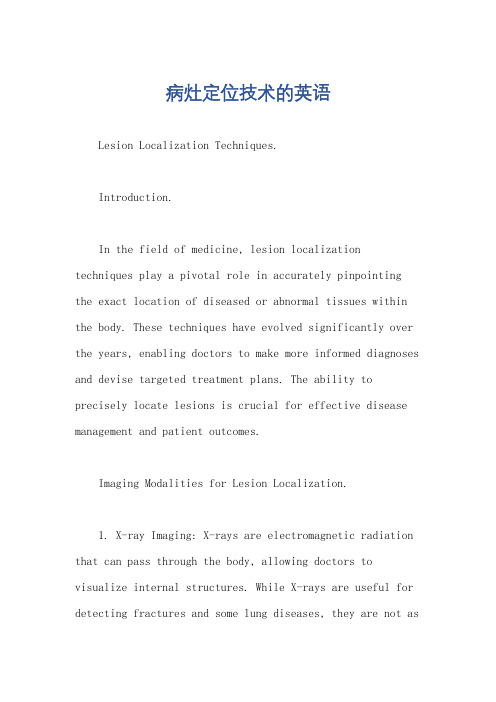
病灶定位技术的英语Lesion Localization Techniques.Introduction.In the field of medicine, lesion localization techniques play a pivotal role in accurately pinpointing the exact location of diseased or abnormal tissues within the body. These techniques have evolved significantly over the years, enabling doctors to make more informed diagnoses and devise targeted treatment plans. The ability to precisely locate lesions is crucial for effective disease management and patient outcomes.Imaging Modalities for Lesion Localization.1. X-ray Imaging: X-rays are electromagnetic radiation that can pass through the body, allowing doctors to visualize internal structures. While X-rays are useful for detecting fractures and some lung diseases, they are not assensitive as other imaging modalities for lesion localization.2. Ultrasound Imaging: Ultrasound uses sound waves to create images of internal organs. It is particularly useful for lesion localization in pregnant women, as it can safely visualize the fetus. It is also commonly used in the breast and thyroid.3. Computed Tomography (CT) Scans: CT scans combine multiple X-ray images taken from different angles to create cross-sectional views of the body. This technique is highly accurate for lesion localization and can detect even small abnormalities.4. Magnetic Resonance Imaging (MRI): MRI uses strong magnets and radio waves to generate detailed images of internal structures. It is excellent for soft tissue visualization and lesion localization, particularly in the brain and joints.5. Positron Emission Tomography (PET) Scans: PET scansdetect gamma rays emitted by radiolabeled substances injected into the patient. This technique is useful for lesion localization in cancer patients, as it can show tumor activity and spread.Advanced Lesion Localization Techniques.1. Fusion Imaging: This technique combines the data from multiple imaging modalities to create a single, comprehensive image. For example, PET-CT fusion imaging combines the functional information from a PET scan with the anatomical detail from a CT scan, improving lesion localization accuracy.2. Image-guided Interventions: These procedures use real-time imaging to guide surgical or therapeutic interventions. Examples include biopsy procedures, where a needle is guided to the lesion using ultrasound or CT imaging, or radiation therapy, where high-energy beams are precisely targeted to the lesion.3. Artificial Intelligence (AI) and Machine Learning:These technologies are revolutionizing lesion localization by enabling computers to analyze vast amounts of imaging data and detect patterns that may indicate disease. AI-powered algorithms can assist doctors in identifying lesions and even predicting their behavior.Conclusion.The evolution of lesion localization techniques has been transformative in medicine, enabling doctors to diagnose and treat patients more effectively. From traditional imaging modalities to advanced fusion imaging and AI-powered analysis, these techniques are constantly evolving to improve patient outcomes. As technology continues to advance, so will our ability to pinpoint and treat lesions with precision and accuracy.。
somatom definition flash简介 -回复
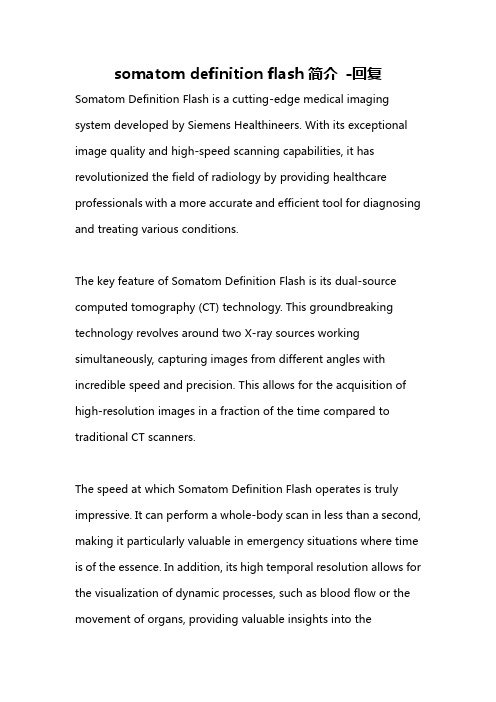
somatom definition flash简介-回复Somatom Definition Flash is a cutting-edge medical imaging system developed by Siemens Healthineers. With its exceptional image quality and high-speed scanning capabilities, it has revolutionized the field of radiology by providing healthcare professionals with a more accurate and efficient tool for diagnosing and treating various conditions.The key feature of Somatom Definition Flash is its dual-source computed tomography (CT) technology. This groundbreaking technology revolves around two X-ray sources working simultaneously, capturing images from different angles with incredible speed and precision. This allows for the acquisition of high-resolution images in a fraction of the time compared to traditional CT scanners.The speed at which Somatom Definition Flash operates is truly impressive. It can perform a whole-body scan in less than a second, making it particularly valuable in emergency situations where time is of the essence. In addition, its high temporal resolution allows for the visualization of dynamic processes, such as blood flow or the movement of organs, providing valuable insights into thefunctioning of the human body.One of the main advantages of Somatom Definition Flash is its ability to minimize radiation exposure while maintaining excellent image quality. The dual-source technology enables the scanner to deliver precise and reliable results using a lower dose of radiation. This is especially important for patients who require frequent imaging or for children who are more sensitive to radiation.The exceptional image quality produced by Somatom Definition Flash enhances diagnostic accuracy and facilitates the detection of subtle abnormalities. Its advanced image reconstruction algorithms ensure clear and detailed images, minimizing the need for repeat examinations and reducing patient discomfort. This is particularly beneficial in cases where early and accurate diagnosis can greatly impact the outcome of treatment.The versatility of Somatom Definition Flash is another distinguishing feature. It offers various imaging options tailored to different clinical needs, such as angiography, cardiac imaging, neurology, oncology, and musculoskeletal imaging. This versatility enables healthcare professionals to effectively address a widerange of conditions, leading to more targeted and personalized treatments.Another remarkable aspect of Somatom Definition Flash is its user-friendly interface and advanced automation capabilities. The system is designed to streamline the imaging process, optimizing workflow and reducing the time required to obtain results. It also includes advanced post-processing software that allows physicians to manipulate the acquired images for accurate diagnosis and treatment planning.In conclusion, Somatom Definition Flash is a state-of-the-art medical imaging system that has revolutionized the field of radiology. Its dual-source technology, high-speed scanning capabilities, exceptional image quality, and low radiation dose make it a valuable tool for healthcare professionals in diagnosing and treating various conditions. With its versatile imaging options and user-friendly interface, Somatom Definition Flash enhances diagnostic accuracy, improves patient outcomes, and transforms the way medical imaging is conducted.。
大数据技术的作文英语
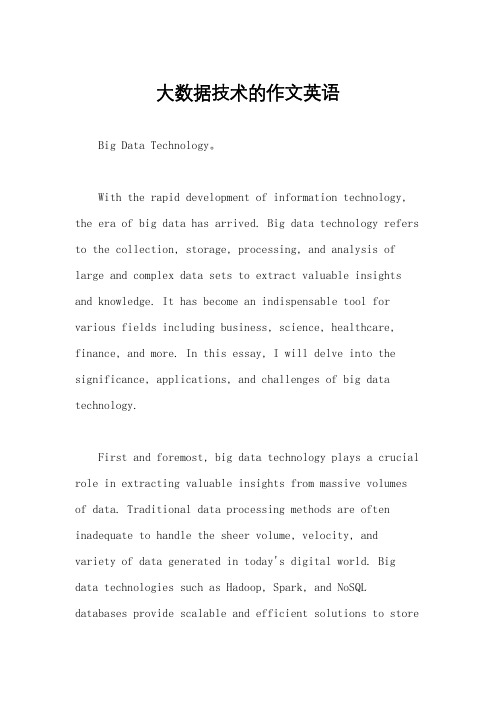
大数据技术的作文英语Big Data Technology。
With the rapid development of information technology, the era of big data has arrived. Big data technology refers to the collection, storage, processing, and analysis of large and complex data sets to extract valuable insights and knowledge. It has become an indispensable tool for various fields including business, science, healthcare, finance, and more. In this essay, I will delve into the significance, applications, and challenges of big data technology.First and foremost, big data technology plays a crucial role in extracting valuable insights from massive volumes of data. Traditional data processing methods are often inadequate to handle the sheer volume, velocity, andvariety of data generated in today's digital world. Big data technologies such as Hadoop, Spark, and NoSQL databases provide scalable and efficient solutions to storeand process vast amounts of data. These technologies enable organizations to gain deeper insights into customer behavior, market trends, and operational efficiency.One of the significant applications of big data technology is in business and marketing. Companies can analyze customer data to understand their preferences, purchasing behavior, and sentiment towards products or services. This enables personalized marketing campaigns, targeted advertising, and product recommendations, leading to better customer engagement and increased sales. For example, e-commerce giant Amazon utilizes big dataanalytics to recommend products based on users' browsing and purchasing history, resulting in a significant increase in sales revenue.Moreover, big data technology has revolutionized healthcare by facilitating data-driven decision-making and personalized medicine. Healthcare providers can analyze electronic health records, medical imaging, and genomic data to identify patterns, diagnose diseases, and recommend personalized treatment plans. This leads to better patientoutcomes, reduced healthcare costs, and improved population health management. For instance, IBM's Watson Health platform leverages big data analytics to assist healthcare professionals in diagnosing and treating cancer patients more effectively.Furthermore, big data technology has immense potential in scientific research and discovery. Scientists can analyze large datasets generated from experiments, simulations, and observations to uncover new insights and knowledge across various disciplines. This includes areas such as climate modeling, genomics, particle physics, and astronomy. For example, the Large Hadron Collider (LHC) generates petabytes of data from particle collisions, which are analyzed using big data techniques to discover new particles and understand the fundamental laws of physics.Despite its numerous benefits, big data technology also poses several challenges. One of the primary challenges is data privacy and security. As large volumes of sensitive data are collected and stored, there is a risk of data breaches, unauthorized access, and misuse of personalinformation. Ensuring data privacy and compliance with regulations such as GDPR (General Data Protection Regulation) and HIPAA (Health Insurance Portability and Accountability Act) is crucial to maintain trust and integrity in the use of big data.Another challenge is the need for skilled professionals proficient in big data technologies and data analytics. There is a growing demand for data scientists, data engineers, and data analysts who can manage and analyze large datasets effectively. However, there is a shortage of talent with the necessary skills and expertise, leading to a competitive job market and recruitment challenges for organizations.In conclusion, big data technology has revolutionized the way we collect, store, process, and analyze data, enabling unprecedented insights and opportunities across various industries. From business and marketing to healthcare and scientific research, big data technology has transformed the way we make decisions, innovate, and solve complex problems. However, it also poses challenges such asdata privacy, security, and skills shortage. Addressing these challenges will be crucial in harnessing the full potential of big data technology for the benefit of society.。
科学作文医学影像与人工智能

科学作文医学影像与人工智能英文回答:Medical imaging plays a crucial role in the field of medicine as it allows healthcare professionals to visualize and diagnose various medical conditions. With the advancements in technology, particularly in the field of artificial intelligence (AI), the integration of medical imaging and AI has the potential to revolutionize healthcare.One of the main benefits of combining medical imaging and AI is the improvement in accuracy and efficiency of diagnoses. AI algorithms can analyze medical images and detect abnormalities or patterns that may be missed by human radiologists. This can lead to earlier detection of diseases and more accurate diagnoses, ultimately improving patient outcomes.For example, AI-powered algorithms can analyzemammograms and identify early signs of breast cancer. This can help in detecting breast cancer at an early stage when it is more treatable. Similarly, AI algorithms can analyze brain scans and identify signs of stroke or other neurological conditions, allowing for prompt intervention and treatment.Another advantage of using AI in medical imaging is the ability to automate repetitive tasks. Radiologists often spend a significant amount of time reviewing and analyzing medical images. AI algorithms can automate these tasks, allowing radiologists to focus on more complex cases and providing faster results to patients.Furthermore, AI can assist in the development of personalized treatment plans. By analyzing medical images and patient data, AI algorithms can predict how a patient may respond to a particular treatment. This can help healthcare professionals in making informed decisions about the most effective treatment options for individual patients.In addition to diagnosis and treatment, AI can also be utilized in medical imaging for research purposes. AI algorithms can analyze large datasets of medical images, identifying patterns or correlations that may not be apparent to human researchers. This can lead to new discoveries and advancements in the understanding ofvarious diseases.Overall, the integration of medical imaging and AI has the potential to greatly enhance healthcare. By improving accuracy and efficiency in diagnoses, automating repetitive tasks, assisting in personalized treatment plans, andaiding in research, AI can revolutionize the field of medicine and improve patient outcomes.中文回答:医学影像在医学领域中起着至关重要的作用,它使医护人员能够可视化和诊断各种疾病。
高一数字世界英语阅读理解25题
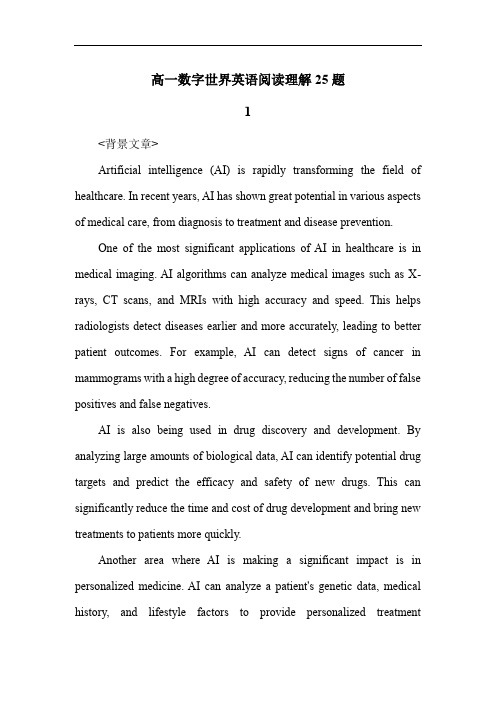
高一数字世界英语阅读理解25题1<背景文章>Artificial intelligence (AI) is rapidly transforming the field of healthcare. In recent years, AI has shown great potential in various aspects of medical care, from diagnosis to treatment and disease prevention.One of the most significant applications of AI in healthcare is in medical imaging. AI algorithms can analyze medical images such as X-rays, CT scans, and MRIs with high accuracy and speed. This helps radiologists detect diseases earlier and more accurately, leading to better patient outcomes. For example, AI can detect signs of cancer in mammograms with a high degree of accuracy, reducing the number of false positives and false negatives.AI is also being used in drug discovery and development. By analyzing large amounts of biological data, AI can identify potential drug targets and predict the efficacy and safety of new drugs. This can significantly reduce the time and cost of drug development and bring new treatments to patients more quickly.Another area where AI is making a significant impact is in personalized medicine. AI can analyze a patient's genetic data, medical history, and lifestyle factors to provide personalized treatmentrecommendations. This can improve the effectiveness of treatment and reduce the risk of adverse reactions.However, the use of AI in healthcare also faces several challenges. One of the main challenges is data privacy and security. Medical data is highly sensitive and must be protected from unauthorized access and use. Another challenge is the lack of transparency and interpretability of AI algorithms. It can be difficult for doctors and patients to understand how AI makes decisions, which can lead to mistrust and resistance.Despite these challenges, the potential benefits of AI in healthcare are enormous. As technology continues to advance, we can expect to see even more innovative applications of AI in the field of medicine.1. What is one of the main applications of AI in healthcare mentioned in the passage?A. Medical research.B. Medical imaging.C. Hospital management.D. Patient care.答案:B。
advanced optical materials分区
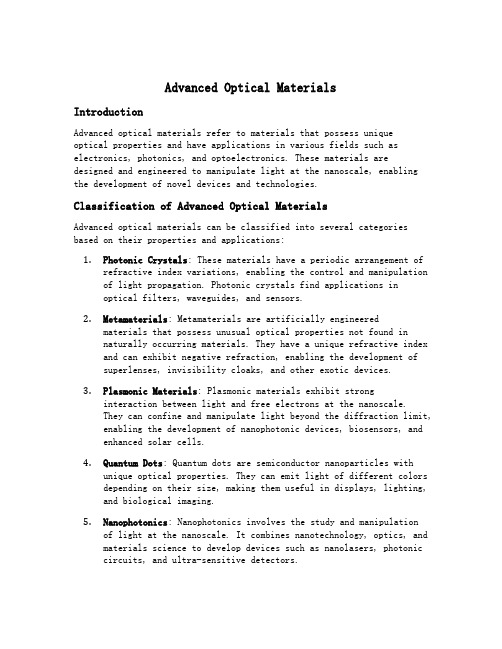
Advanced Optical MaterialsIntroductionAdvanced optical materials refer to materials that possess uniqueoptical properties and have applications in various fields such as electronics, photonics, and optoelectronics. These materials are designed and engineered to manipulate light at the nanoscale, enabling the development of novel devices and technologies.Classification of Advanced Optical MaterialsAdvanced optical materials can be classified into several categories based on their properties and applications:1.Photonic Crystals: These materials have a periodic arrangement ofrefractive index variations, enabling the control and manipulation of light propagation. Photonic crystals find applications inoptical filters, waveguides, and sensors.2.Metamaterials: Metamaterials are artificially engineeredmaterials that possess unusual optical properties not found innaturally occurring materials. They have a unique refractive index and can exhibit negative refraction, enabling the development ofsuperlenses, invisibility cloaks, and other exotic devices.3.Plasmonic Materials: Plasmonic materials exhibit stronginteraction between light and free electrons at the nanoscale.They can confine and manipulate light beyond the diffraction limit, enabling the development of nanophotonic devices, biosensors, andenhanced solar cells.4.Quantum Dots: Quantum dots are semiconductor nanoparticles withunique optical properties. They can emit light of different colors depending on their size, making them useful in displays, lighting, and biological imaging.5.Nanophotonics: Nanophotonics involves the study and manipulationof light at the nanoscale. It combines nanotechnology, optics, and materials science to develop devices such as nanolasers, photoniccircuits, and ultra-sensitive detectors.6.Optical Fibers: Optical fibers are thin, flexible strands ofglass or plastic that can transmit light over long distances withminimal loss. They are used in telecommunications, medical imaging, and sensing applications.Applications of Advanced Optical MaterialsAdvanced optical materials have a wide range of applications across various fields:rmation Technology: Advanced optical materials are crucialfor the development of faster and more efficient data storage andcommunication technologies. Photonic crystals and metamaterialsare used in optical data storage, high-speed optical communication, and optical computing.2.Sensing and Imaging: Advanced optical materials play a vital rolein sensing and imaging technologies. Plasmonic materials andquantum dots are used in biosensors for detecting biologicalmolecules and in medical imaging for improved contrast andresolution.3.Energy: Advanced optical materials are used in solar cells toenhance light absorption and improve energy conversion efficiency.They are also used in light-emitting diodes (LEDs) for efficientand high-quality lighting.4.Optical Devices: Advanced optical materials enable thedevelopment of compact and efficient optical devices.Nanophotonics and photonic crystals are used in the fabrication of nanolasers, photonic integrated circuits, and ultra-sensitivedetectors.5.Biotechnology: Advanced optical materials have applications inbiotechnology and medicine. Quantum dots and plasmonic materialsare used for cellular imaging, drug delivery, and cancer therapy.Future OutlookThe field of advanced optical materials continues to evolve rapidly, driven by advancements in nanotechnology, materials science, and optics. Future research and development efforts are focused on:1.Enhancing Performance: Researchers are working on improving theoptical properties of advanced materials, such as increasing their efficiency, stability, and tunability.2.Integration and Miniaturization: The integration of advancedoptical materials into compact and multifunctional devices is amajor focus of ongoing research. This includes the development of on-chip photonic circuits and nanoscale optical devices.3.Biomedical Applications: Advanced optical materials hold greatpromise in biotechnology and medicine. Ongoing research aims todevelop targeted drug delivery systems, high-resolution imagingtechniques, and optical sensors for disease detection.4.Emerging Technologies: The field of advanced optical materials isalso exploring new technologies such as plasmonics, quantumphotonics, and 2D materials for novel applications incommunications, sensing, and computing.In conclusion, advanced optical materials offer immense potential for the development of next-generation devices and technologies. Their unique optical properties and versatile applications make them a vital area of research and innovation. Continued advancements in this field will drive progress in various sectors, from information technology to healthcare.。
成像技术英文作文

成像技术英文作文英文:Imaging technology has revolutionized the way we see and understand the world around us. From medical imaging to satellite imagery, there are a wide range of applications for imaging technology. In this essay, I will discuss the different types of imaging technology and their uses.One of the most common types of imaging technology is X-ray imaging. This technology is used in medical imaging to see inside the human body. X-rays are able to penetrate soft tissue and bone, allowing doctors to see inside the body and diagnose medical conditions. Another type of medical imaging is magnetic resonance imaging (MRI). This technology uses magnetic fields to create detailed images of the body's internal structures. MRIs are often used to diagnose conditions such as tumors, infections, and injuries.In addition to medical imaging, there are also manyother applications for imaging technology. For example, satellite imagery is used to monitor weather patterns and track natural disasters. This technology is also used in agriculture to monitor crop growth and identify areas that need more water or fertilizer. Another application of imaging technology is in the field of art conservation. Imaging technology can be used to analyze paintings and identify areas of damage or deterioration.Overall, imaging technology has had a significantimpact on many different fields. From medicine toagriculture to art conservation, there are a wide range of applications for this technology.中文:成像技术已经彻底改变了我们看待和理解周围世界的方式。
介绍全息技术英语作文

介绍全息技术英语作文Title: Exploring the World of Holographic Technology。
In today's digital era, holographic technology has emerged as a groundbreaking innovation that revolutionizes the way we perceive and interact with the world around us. From entertainment and education to healthcare and manufacturing, holographic technology holds immensepotential in various fields. This essay delves into the intricacies of holographic technology, its applications,and its impact on society.Holography, derived from the Greek words "holos" (whole) and "graphein" (to write), is the science and practice of creating three-dimensional images called holograms. Unlike traditional photographs, which capture only the intensityof light, holograms record both the intensity and phase of light waves, providing a more realistic and immersivevisual experience.One of the most remarkable aspects of holographic technology is its versatility in applications. In the realm of entertainment, holograms have transcended the boundaries of traditional performances, enabling artists to stage virtual concerts and resurrect deceased icons for awe-inspiring performances. These holographic concerts not only captivate audiences but also preserve the legacy of legendary performers for future generations.Moreover, holographic technology has revolutionized the way we perceive visual content in education and training. By creating lifelike simulations and virtual models, educators can enhance learning experiences and facilitate better understanding of complex subjects such as anatomy, physics, and architecture. Medical students, for instance, can explore detailed holographic representations of the human body, allowing them to study anatomy in unprecedented detail.In the field of healthcare, holographic technology holds immense promise for medical imaging and diagnostics. Holographic displays can provide physicians withinteractive 3D representations of patient data, enabling more accurate diagnoses and personalized treatment plans. Furthermore, surgeons can use holographic overlays during procedures to visualize internal structures in real-time, leading to improved surgical outcomes and patient safety.Beyond entertainment and healthcare, holographic technology is poised to revolutionize communication and collaboration. With the advent of holographic telepresence, individuals can interact with remote counterparts as if they were physically present, transcending geographical barriers and fostering seamless collaboration across borders. This technology has the potential to reshape the way we conduct business, hold meetings, and connect with others on a global scale.In the realm of design and manufacturing, holographic technology is driving innovation and efficiency. Designers and engineers can use holographic displays to visualize and manipulate 3D models with unparalleled precision, accelerating the product development process and reducing time-to-market. Additionally, holographic prototypingenables manufacturers to test product designs in virtual environments, minimizing costly errors and optimizing production workflows.Despite its myriad benefits, holographic technology also presents ethical and societal challenges that warrant careful consideration. As holograms become increasingly indistinguishable from reality, questions arise regarding the authenticity of digital content and the potential for misuse, such as the creation of deceptive or misleading visuals. Moreover, concerns about privacy and data security may arise as holographic telepresence becomes more prevalent, raising questions about the boundaries between public and private spaces.In conclusion, holographic technology represents a paradigm shift in how we perceive and interact with the world, offering unprecedented opportunities for innovation across various industries. From entertainment and education to healthcare and manufacturing, holographic technology is poised to reshape the way we live, work, and communicate in the digital age. However, as we embrace the potential ofholographic technology, it is essential to address ethical considerations and ensure that its benefits are harnessed responsibly for the betterment of society.。
人工智能在医疗方面的应用英语作文
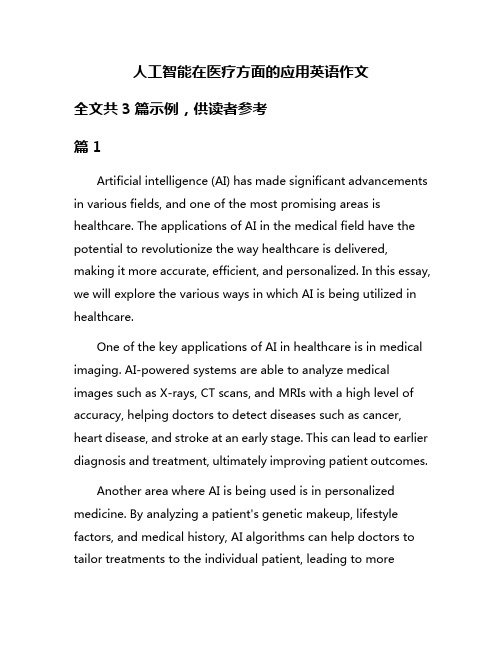
人工智能在医疗方面的应用英语作文全文共3篇示例,供读者参考篇1Artificial intelligence (AI) has made significant advancements in various fields, and one of the most promising areas is healthcare. The applications of AI in the medical field have the potential to revolutionize the way healthcare is delivered, making it more accurate, efficient, and personalized. In this essay, we will explore the various ways in which AI is being utilized in healthcare.One of the key applications of AI in healthcare is in medical imaging. AI-powered systems are able to analyze medical images such as X-rays, CT scans, and MRIs with a high level of accuracy, helping doctors to detect diseases such as cancer, heart disease, and stroke at an early stage. This can lead to earlier diagnosis and treatment, ultimately improving patient outcomes.Another area where AI is being used is in personalized medicine. By analyzing a patient's genetic makeup, lifestyle factors, and medical history, AI algorithms can help doctors to tailor treatments to the individual patient, leading to moreeffective and efficient care. Personalized medicine has the potential to revolutionize the way we treat diseases, moving away from the one-size-fits-all approach towards a more targeted and precise form of treatment.AI is also being used to improve patient care and outcomes through remote monitoring and telehealth. By using wearable devices and sensors, AI algorithms can monitor a patient's vital signs and alert healthcare providers to any abnormalities in real-time. This enables early intervention and can prevent serious health complications. Telehealth, on the other hand, allows patients to consult with healthcare providers remotely, reducing the need for in-person visits and making healthcare more accessible and convenient.In addition to these applications, AI is also being used to streamline administrative processes in healthcare, such as scheduling appointments, billing, and managing patient records. AI-powered systems are able to automate these tasks, freeing up healthcare providers to focus on patient care. This can lead to increased efficiency, reduced costs, and improved patient satisfaction.Despite the many benefits of AI in healthcare, there are also challenges and concerns that need to be addressed. One of themain concerns is data privacy and security, as AI systems collect and analyze a large amount of sensitive patient information. It is important to implement robust security measures to protect patient data and ensure compliance with privacy regulations.Another challenge is the potential for bias in AI algorithms. If these algorithms are trained on biased data, they may perpetuate existing inequalities in healthcare. To address this issue, it is important to ensure that AI algorithms are trained on diverse and representative data sets.In conclusion, AI has the potential to transform healthcare by improving diagnosis, treatment, and patient care. By leveraging the power of AI, we can make healthcare more accurate, efficient, and personalized, ultimately improving patient outcomes and reducing costs. However, it is important to address the challenges and concerns associated with AI in healthcare to ensure that these technologies are used responsibly and ethically.篇2Artificial intelligence (AI) has revolutionized many industries, and healthcare is no exception. The application of AI in healthcare has the potential to transform the way medicaldiagnoses are made, treatments are administered, and patient outcomes are improved. In this essay, we will explore the various ways in which AI is being utilized in the field of healthcare.One of the most significant applications of AI in healthcare is in medical imaging. AI algorithms are able to analyze medical images, such as X-rays, MRIs, and CT scans, with a level of accuracy and speed that far surpasses human capabilities. This allows for the early detection of diseases such as cancer, cardiovascular disease, and neurological disorders, leading to earlier intervention and better outcomes for patients.In addition to medical imaging, AI is also being used in the development of personalized treatment plans for patients. By analyzing large amounts of patient data, AI algorithms can identify patterns and make predictions about how a particular patient will respond to a specific treatment. This allows for more targeted and effective treatments, reducing the likelihood of adverse reactions and improving patient outcomes.Another area where AI is making a significant impact in healthcare is in the field of drug discovery. Developing new drugs is a highly complex and time-consuming process, but AI has the potential to streamline this process by identifying potential drug candidates more quickly and accurately. Byanalyzing vast amounts of data, AI algorithms can predict the efficacy and safety of new drugs, speeding up the development process and bringing new treatments to market faster.AI is also being used to improve the efficiency of healthcare systems. By analyzing patient data and healthcare workflows, AI algorithms can identify areas where inefficiencies exist and suggest ways to streamline processes and reduce costs. This can lead to improved patient care, reduced wait times, and better utilization of resources.In addition to these applications, AI is also being used to improve the quality of healthcare education. AI-powered virtual reality simulations are being used to train medical students and professionals, allowing them to practice complex procedures in a realistic and risk-free environment. This not only improves the skills of healthcare providers but also increases patient safety by reducing the likelihood of medical errors.While the potential benefits of AI in healthcare are vast, there are also challenges and ethical considerations that need to be addressed. Issues such as data privacy, algorithm bias, and the potential impact on jobs in the healthcare industry need to be carefully considered as AI continues to be integrated into healthcare systems.In conclusion, the application of AI in healthcare has the potential to revolutionize the way medical care is delivered, improving patient outcomes, reducing costs, and increasing efficiency. As AI technology continues to advance, it is essential that healthcare providers, policymakers, and researchers work together to harness the power of AI in a way that maximizes its benefits while minimizing potential risks. By doing so, we can create a future in which AI enables us to provide high-quality, personalized healthcare to all.篇3Artificial intelligence has been making significant strides in the field of healthcare, revolutionizing the way diseases are diagnosed, treated, and managed. With the ability to process and analyze vast amounts of data quickly and accurately, AI has the potential to improve patient outcomes, reduce healthcare costs, and ultimately save lives.One of the most promising applications of AI in healthcare is in medical imaging. By using machine learning algorithms, AI can analyze medical images such as X-rays, MRIs, and CT scans to detect abnormalities or identify patterns that may be indicative of a disease. This can help radiologists make more accurate diagnoses and provide better treatment options for patients.Another important use of AI in healthcare is in personalized medicine. By analyzing a patient's genetic information, medical history, and other relevant data, AI can help doctors develop personalized treatment plans that are tailored to the individual patient's needs. This can lead to more effective treatments, fewer side effects, and better outcomes for patients.AI can also be used to assist in surgery, by providingreal-time feedback to surgeons and helping them navigate complex procedures more accurately. By using AI-powered surgical robots, surgeons can perform minimally invasive surgeries with greater precision and control, leading to faster recovery times and better outcomes for patients.In addition to improving patient care, AI can also help healthcare providers become more efficient and cost-effective. By automating routine tasks such as scheduling appointments, processing paperwork, and managing inventory, AI can free up healthcare professionals to focus on more important tasks and improve the overall efficiency of healthcare systems.Despite its many benefits, the use of AI in healthcare also raises ethical and privacy concerns. Patients may be worried about the security of their personal data and the potential for AI to make mistakes or misdiagnose conditions. It is important forhealthcare providers to address these concerns and ensure that AI is used responsibly and ethically.Overall, the use of AI in healthcare has the potential to revolutionize the way diseases are diagnosed and treated, leading to better patient outcomes, lower healthcare costs, and improved efficiency in healthcare systems. As AI continues to advance and evolve, it will be crucial for healthcare providers to embrace this technology and harness its potential to benefit patients and society as a whole.。
医学改变生活的英语作文

医学改变生活的英语作文The Transformative Power of Medicine in Our Lives.The field of medicine has undergone remarkable transformations in recent decades, revolutionizing the way we understand, diagnose, and treat a wide range of health conditions. The impact of these advancements is profound, extending far beyond the hospital walls to encompass every aspect of our lives. In this essay, I will explore the ways in which medicine has transformed our lives, focusing on areas such as prevention, diagnosis, treatment, and the broader social implications of these changes.Prevention: Shifting the Focus to Wellness.One of the most significant ways medicine has changed our lives is in the realm of prevention. Traditionally, medicine has focused primarily on treating diseases and conditions after they have occurred. However, recent advancements in fields like genetics, nutrition, andepidemiology have allowed us to shift our focus towards preventing these conditions from developing in the first place.For example, we now have a much better understanding of the role genetics plays in certain diseases, allowing us to identify individuals who may be at risk for certain conditions and take proactive measures to mitigate those risks. Similarly, the rise of personalized nutrition and fitness plans has enabled us to tailor our lifestyle choices to our unique biological needs, promoting overall wellness and reducing the likelihood of developing chronic diseases.Diagnosis: The Role of Technology.Advancements in technology have also revolutionized the diagnostic process. The development of highly sensitive imaging techniques like MRI and CT scans has allowed doctors to detect diseases and conditions with unprecedented accuracy. The use of artificial intelligence and machine learning in diagnostics is furtherrevolutionizing the field, enabling computers to analyze vast amounts of data and identify patterns that mayindicate the presence of a disease.These technological advancements have not only improved the accuracy of diagnoses but have also made the process more efficient and convenient for patients. For instance, remote diagnosis using telemedicine has become increasingly common, allowing individuals to consult with doctors remotely and receive prompt treatment without having to travel to a medical facility.Treatment: Innovations in Therapeutics.The field of therapeutics has also seen remarkable advancements in recent years. The development of targeted therapies and precision medicine has allowed doctors to tailor treatment plans to the individual characteristics of each patient, improving outcomes and reducing the risk of adverse side effects.In addition, the emergence of immunotherapy and genetherapy offers new hope for treating a wide range of conditions that were previously considered incurable. These innovative treatment modalities have the potential to revolutionize medicine, transforming the way we approach diseases and conditions that have long been a challenge to treat effectively.Social Implications.The transformations in medicine have had profoundsocial implications as well. The improved lifespan and quality of life resulting from medical advancements have had a significant impact on society, enabling individuals to live longer, healthier lives.However, these changes have also presented new challenges, such as the rise of chronic diseases and the associated economic and social costs. Addressing these challenges requires a comprehensive approach that involves not only medical professionals but also policy makers, educators, and the community at large.Conclusion.In conclusion, the transformative power of medicine in our lives is indisputable. The advancements in prevention, diagnosis, and treatment have not only improved our health and well-being but have also reshaped the social and economic landscape. As we look towards the future, it is clear that medicine will continue to play a crucial role in shaping our lives and the world we live in. It is imperative that we continue to invest in medical research and education, ensuring that the benefits of these advancements are accessible to all.。
医学科研目标及计划
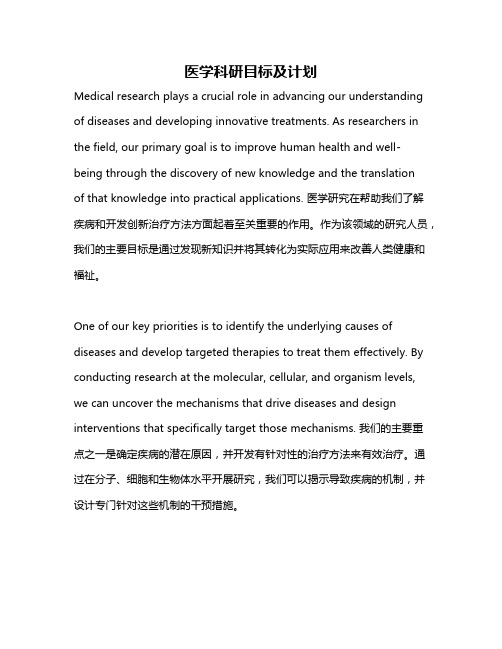
医学科研目标及计划Medical research plays a crucial role in advancing our understanding of diseases and developing innovative treatments. As researchers in the field, our primary goal is to improve human health and well-being through the discovery of new knowledge and the translationof that knowledge into practical applications. 医学研究在帮助我们了解疾病和开发创新治疗方法方面起着至关重要的作用。
作为该领域的研究人员,我们的主要目标是通过发现新知识并将其转化为实际应用来改善人类健康和福祉。
One of our key priorities is to identify the underlying causes of diseases and develop targeted therapies to treat them effectively. By conducting research at the molecular, cellular, and organism levels, we can uncover the mechanisms that drive diseases and design interventions that specifically target those mechanisms. 我们的主要重点之一是确定疾病的潜在原因,并开发有针对性的治疗方法来有效治疗。
通过在分子、细胞和生物体水平开展研究,我们可以揭示导致疾病的机制,并设计专门针对这些机制的干预措施。
生物识别技术英文作文
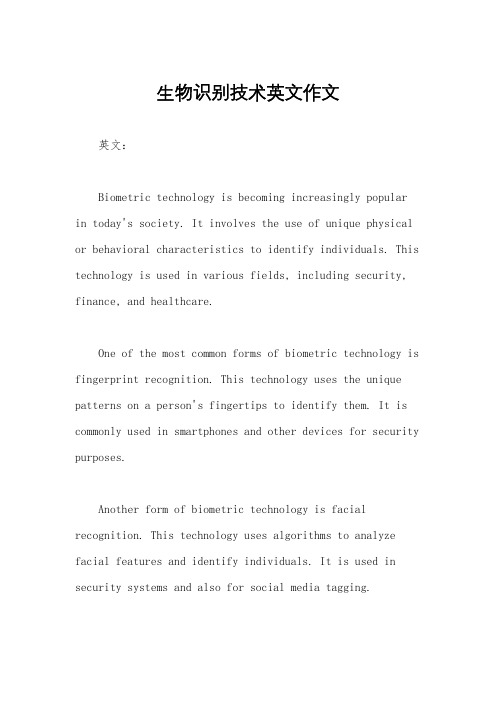
生物识别技术英文作文英文:Biometric technology is becoming increasingly popular in today's society. It involves the use of unique physical or behavioral characteristics to identify individuals. This technology is used in various fields, including security, finance, and healthcare.One of the most common forms of biometric technology is fingerprint recognition. This technology uses the unique patterns on a person's fingertips to identify them. It is commonly used in smartphones and other devices for security purposes.Another form of biometric technology is facial recognition. This technology uses algorithms to analyze facial features and identify individuals. It is used in security systems and also for social media tagging.Voice recognition is another form of biometric technology. It uses the unique characteristics of aperson's voice to identify them. This technology is commonly used in phone systems and other voice-activated devices.One of the benefits of biometric technology is its accuracy. It is difficult to fake or replicate someone's unique physical or behavioral characteristics. This makes it a reliable form of identification.However, there are also concerns about privacy and security. Biometric data is highly personal and sensitive, and there is a risk of it being stolen or misused. It is important for companies and organizations to have strong security measures in place to protect this data.Overall, biometric technology has the potential to revolutionize the way we identify individuals. It offers a high level of accuracy and convenience, but also requires careful consideration of privacy and security issues.中文:生物识别技术在当今社会中越来越受欢迎。
FLIR 高性能冷凝式热成像相机技术说明书
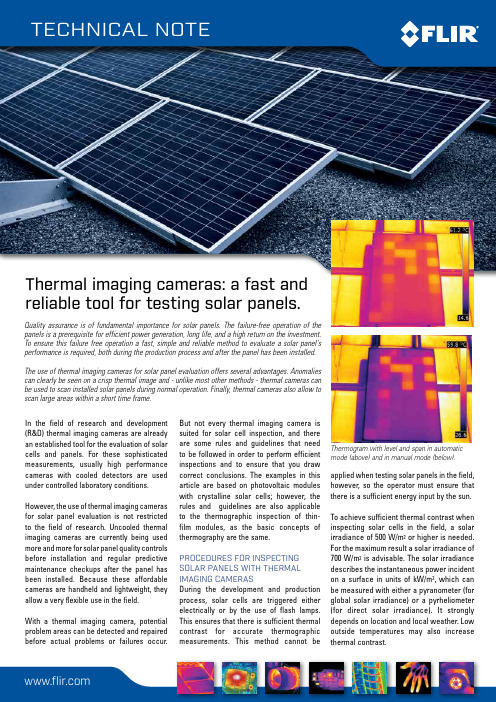
TECHNICAL NOTEIn the field of research and development (R&D) thermal imaging cameras are already an established tool for the evaluation of solar cells and panels. For these sophisticated measurements, usually high performance cameras with cooled detectors are used under controlled laboratory conditions.However, the use of thermal imaging cameras for solar panel evaluation is not restricted to the field of research. Uncooled thermal imaging cameras are currently being used more and more for solar panel quality controls before installation and regular predictive maintenance checkups after the panel has been installed. Because these affordable cameras are handheld and lightweight, they allow a very flexible use in the field.With a thermal imaging camera, potential problem areas can be detected and repaired before actual problems or failures occur. But not every thermal imaging camera is suited for solar cell inspection, and there are some rules and guidelines that needto be followed in order to perform efficient inspections and to ensure that you drawcorrect conclusions. The examples in this article are based on photovoltaic modules with crystalline solar cells; however, the rules and guidelines are also applicableto the thermographic inspection of thin-film modules, as the basic concepts of thermography are the same.PROCEDURES FOR INSPECTING SOLAR PANELS WITH THERMAL IMAGING CAMERAS During the development and production process, solar cells are triggered either electrically or by the use of flash lamps. This ensures that there is sufficient thermal contrast for accurate thermographic measurements. This method cannot be Thermal imaging cameras: a fast and reliable tool for testing solar panels.applied when testing solar panels in the field, however, so the operator must ensure that there is a sufficient energy input by the sun.To achieve sufficient thermal contrast when inspecting solar cells in the field, a solar irradiance of 500 W/m 2 or higher is needed. For the maximum result a solar irradiance of 700 W/m 2 is advisable. The solar irradiance describes the instantaneous power incident on a surface in units of kW/m 2, which can be measured with either a pyranometer (forglobal solar irradiance) or a pyrheliometer (for direct solar irradiance). It stronglydepends on location and local weather. Low outside temperatures may also increase thermal contrast.Quality assurance is of fundamental importance for solar panels. The failure-free operation of the panels is a prerequisite for efficient power generation, long life, and a high return on the investment. To ensure this failure free operation a fast, simple and reliable method to evaluate a solar panel's performance is required, both during the production process and after the panel has been installed. The use of thermal imaging cameras for solar panel evaluation offers several advantages. Anomalies can clearly be seen on a crisp thermal image and - unlike most other methods - thermal cameras can be used to scan installed solar panels during normal operation. Finally, thermal cameras also allow to scan large areas within a short time frame.Thermogram with level and span in automaticmode (above) and in manual mode (below).TECHNICAL NOTE predictive maintenance inspectionshighest when the camera is perpendicular, and decreases with an increasing angle. A viewing angle of 5–60° is a good compromise (where 0° is perpendicular).LONG DISTANCE OBSERVATIONS It is not always easy to achieve a suitable viewing angle during the measurement set-up. Using a tripod can provide a solution in most cases. In more difficult conditions it might be necessary to use mobile working platforms or even to fly over the solar cells with a helicopter. In these cases, the longer distance from the target can be advantageous, since a larger area can be seen in one pass. To ensure the quality of the thermal image, a thermal imaging camera with an image resolution of at least 320 × 240 pixels, preferably 640 × 480 pixels, should be used for these longer distances.The camera should also have an interchangeable lens, so the operator can switch to a telephoto lens for long distance observations, such as from a helicopter. It is advisable, however, to only use telephoto lenses with thermal imaging cameras that have a high image resolution. Low resolution thermal imaging cameras will be unable to pick up the small thermal details thatindicate solar panel faults in long distance measurements using a telephoto lens.LOOKING AT IT FROM A DIFFERENT PERSPECTIVE In most cases installed photovoltaic modules can also be inspected with a thermal imaging camera from the rear of amodule. This method minimizes interfering reflections from the sun and the clouds. In addition, the temperatures obtained at the back may be higher, as the cell is being measured directly and not through the glass surface.AMBIENT AND MEASUREMENT CONDITIONS When undertaking thermographic inspections, the sky should be clear since clouds reduce solar irradiance and also produce interference through reflections. Informative images can, however, be obtained even with an overcast sky, provided that the thermal imaging camera used is sufficiently sensitive. Calm conditions are desirable, since any airflow on the surface of the solar module will cause convective cooling and thus will reduce the thermal gradient. The cooler the air temperature, the higher the potential thermal contrast. Performing thermographic inspections in the early morning is an option.Another way to enhance thermal contrast is to disconnect the cells from a load, to prevent the flow of current, which allowsheating to occur through solar irradiance alone. A load is then connected, and the cells are observed in the heating phase.Under normal circumstances, however, thesystem should be inspected under standard operating conditions, namely under load. Depending on the type of cell and the kind of fault or failure, measurements under no-load or short-circuit conditions can provide additional information.MEASUREMENT ERRORSMeasurement errors arise primarily due to poor camera positioning and suboptimal ambient and measurement conditions. Typical measurement errors are caused by:• too shallow viewing angle • c hange in solar irradiance over time (due to changes in sky cover, for example)• r eflections (e.g., sun, clouds,surrounding buil d ings of greater height, measurement set-ups)• p artial shadowing (e.g., due to surrounding buildings or other structures).Thermal image made using a FLIR P660 cameraon a flight over a solar farm. (Thermogramcourtesy of Evi Müllers, IMM)In order not to draw false conclusions you need to hold the thermal imaging camera under a correct angle when inspecting solar panels.This thermal image shows large areas withelevated temperatures. Without more information, it is not obvious whether these are thermal anomalies or shadowing/reflections.Thermal image of the back of a solar module taken with a FLIR P660 camera. Its corresponding visual image is shown on the right.For more information visit or contact:FLIR Commercial Systems B.V.Charles Petitweg 214847 NW Breda - Netherlands Phone : +31 (0) 765 79 41 94Fax : +31 (0) 765 79 41 99e-mail :*************T 820228 {E N _u k }_ATECHNICAL NOTEWHAT CAN YOU SEE IN THE THERMAL IMAGEIf parts of the solar panel are hotter than others, the warm areas will show up clearly in the thermal image. Depending on the shape and location, these hot spots and areas can indicate several different faults. If an entire module is warmer thanusual that might indicate interconnection problems. If individual cells or strings of cells are showing up as a hot spot or a warmer ‘patchwork pattern’, the cause can usually be found either in defective bypass diodes, in internal short-circuits, or in a cell mismatch.Shadowing and cracks in cells show up as hot spots or polygonal patches in thethermal image. The temperature rise of a cell or of part of a cell indicates a defective cell or shadowing. Thermal images obtained under load, no-load, and short-circuit conditions should be compared. A comparison of thermal images of the front and rear faces of the module can also give valuable information. Of course, for correct identification of the failure, modules showing anomalies must also be tested electrically and inspected visually.CONCLUSIONSThe thermographic inspection of photovoltaic systems allows the fast localization of potential defects at the cell and module level as well as the detection of possible electrical interconnection problems. The inspections are carried out under normal operating conditions and do not require a system shut down.For correct and informative thermal images, certain conditions and measurement procedures should be observed:• a suitable thermal imaging camera with the right accessories should be used;• s ufficient solar irradiance is required (at least 500 W/m 2 – above 700 W/m 2 preferred);• t he viewing angle must be within the safe margins (between 5° and 60°);• s hadowing and reflections must be prevented.Thermal imaging cameras are primarily used to locate defects. Classification and assessment of the anomalies detected require a sound understanding of solar technology, knowledge of the system inspected, and additional electrical measurements. Proper documentation is, of course, a must, and should contain all inspection conditions, additional measurements, and other relevant information.Inspections with a thermal imagingcamera - starting with the quality control in the installation phase, followed by regular checkups - facilitate complete and simple system condition monitoring. This will help to maintain the solar panels' functionality and to extend their lifetime. Using thermal imaging cameras for solar panel inspections will therefore drastically improve the operating company’s return oninvestment.Table 1: List of typical module errors (Source: ZAE Bayern e.V , “Überprüfung der Qualität von Photovoltaik-Modulen mittels Infrarot-Aufnahmen” ["Quality testing in photovoltaic modules using infrared imaging”], 2007)These red spots indicate modules that are consistently hotter than the rest, indicating faulty connections.This hot spot within one solar cell indicates physical damage within the cell.This thermal image shows an example of the so-called ‘patchwork pattern’, which indicates that this panel has a defective bypass diode.。
nature biomedical engineering文章模板
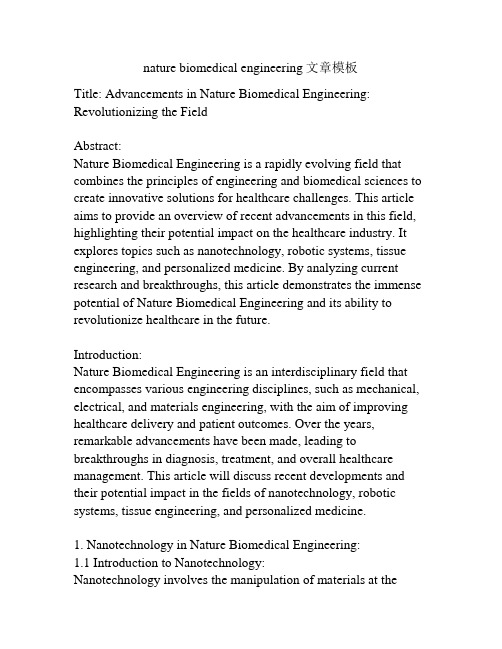
nature biomedical engineering文章模板Title: Advancements in Nature Biomedical Engineering: Revolutionizing the FieldAbstract:Nature Biomedical Engineering is a rapidly evolving field that combines the principles of engineering and biomedical sciences to create innovative solutions for healthcare challenges. This article aims to provide an overview of recent advancements in this field, highlighting their potential impact on the healthcare industry. It explores topics such as nanotechnology, robotic systems, tissue engineering, and personalized medicine. By analyzing current research and breakthroughs, this article demonstrates the immense potential of Nature Biomedical Engineering and its ability to revolutionize healthcare in the future.Introduction:Nature Biomedical Engineering is an interdisciplinary field that encompasses various engineering disciplines, such as mechanical, electrical, and materials engineering, with the aim of improving healthcare delivery and patient outcomes. Over the years, remarkable advancements have been made, leading to breakthroughs in diagnosis, treatment, and overall healthcare management. This article will discuss recent developments and their potential impact in the fields of nanotechnology, robotic systems, tissue engineering, and personalized medicine.1. Nanotechnology in Nature Biomedical Engineering:1.1 Introduction to Nanotechnology:Nanotechnology involves the manipulation of materials at thenanoscale level for various applications. In biomedical engineering, nanotechnology has had a significant impact on drug delivery systems, biosensors, and imaging techniques.1.2 Applications in Drug Delivery:Nanoparticles have shown promise in improving drug solubility, enhancing drug targeting, and reducing systemic side effects. Recent studies have focused on developing nanoparticle-based drug delivery systems for cancer treatment, CNS disorders, and targeted therapy.1.3 Biosensors and Imaging:Nanotechnology has enabled the development of highly sensitive biosensors for the early detection of diseases and monitoring of biomarkers. Additionally, imaging techniques such as nano-imaging and quantum dots have shown potential in improving imaging resolution and accuracy.2. Robotic Systems in Nature Biomedical Engineering:2.1 Surgical Robotics:Robotic systems have revolutionized surgical procedures by providing enhanced precision, improved visualization, and reduced invasiveness. Surgical robots, such as the da Vinci Surgical System, have been widely adopted in procedures like minimally invasive surgery, cardiac surgery, and neurosurgery.2.2 Rehabilitation and Assistive Robots:Robotic systems are increasingly being used for rehabilitation purposes, aiding individuals with disabilities in regaining their motor functions. Assistive robots, including exoskeletons, canassist patients in performing daily activities and improve their quality of life.3. Tissue Engineering in Nature Biomedical Engineering:3.1 Introduction to Tissue Engineering:Tissue engineering involves the fabrication of functional tissues and organs using a combination of cells, biomaterials, and growth factors. This field holds immense potential in regenerative medicine and organ transplantation.3.2 3D Bioprinting:Advancements in 3D bioprinting have allowed for the creation of complex structures and organs. Researchers have successfully bioprinted tissues like cardiac patches, bone, cartilage, and skin, offering new possibilities for organ transplantation in the future.4. Personalized Medicine in Nature Biomedical Engineering:4.1 Biomarkers and Diagnostic Tests:The development of personalized medicine relies on identifying reliable biomarkers and diagnostic tests for early disease detection and prediction of treatment response. Advances in genomics, proteomics, and metabolomics have led to the discovery of numerous biomarkers.4.2 Targeted Therapies:Personalized medicine allows for tailored treatment plans based on an individual's genetic makeup, ensuring maximum efficacy and minimal side effects. Targeted therapies like gene therapy, immunotherapy, and precision medicine are increasingly being used in the clinical setting.Conclusion:Nature Biomedical Engineering is a rapidly evolving field with the potential to transform healthcare as we know it. From nanotechnology to robotic systems, tissue engineering, and personalized medicine, recent advancements have paved the way for innovative solutions in diagnosis, treatment, and healthcare management. Continued research and collaborations in this field will undeniably lead to further breakthroughs, revolutionizing the healthcare industry and improving patient outcomes on a global scale.。
英语作文-农业科学研究和试验发展行业的智能化技术与数字化转型

英语作文-农业科学研究和试验发展行业的智能化技术与数字化转型The integration of intelligent technologies and digital transformation in the field of agricultural science research and experimental development is revolutionizing the way we approach farming and food production. At the heart of this transformation is the utilization of data and advanced analytics to drive decision-making and optimize agricultural practices.Intelligent technologies, such as precision agriculture, leverage sensors, GPS, and big data analytics to monitor crop health, soil conditions, and weather patterns. This allows for more precise application of water, fertilizers, and pesticides, which not only increases crop yields but also reduces environmental impact. For instance, drones equipped with multispectral imaging cameras can assess plant health across vast fields, pinpointing areas that need attention and enabling targeted intervention.Digital transformation in agriculture extends to the management of resources and supply chains. Blockchain technology, for example, provides a secure and transparent way to track the journey of food products from farm to table. This traceability ensures food safety and quality, and also enhances consumer trust. Moreover, it allows farmers to receive fair compensation for their produce by eliminating intermediaries.Artificial intelligence (AI) plays a pivotal role in analyzing agricultural data. Machine learning algorithms can predict crop yields, detect plant diseases, and provide insights into genetic information that can lead to the development of more resilient crop varieties. AI-driven robots are increasingly being used for tasks such as harvesting, weeding, and pollination, thereby addressing labor shortages and improving operational efficiency.The digitalization of agriculture also involves the use of Internet of Things (IoT) devices. These connected devices can automate irrigation systems, climate controls in greenhouses, and feeding mechanisms in livestock management. The data collected fromIoT devices can be used to create predictive models for farm operations, leading to more informed decisions and better resource allocation.Despite the numerous benefits, the adoption of intelligent technologies and digital transformation in agriculture faces challenges. There is a need for significant investment in infrastructure and training for farmers to effectively use these technologies. Additionally, concerns around data privacy and cybersecurity must be addressed to protect sensitive agricultural data.In conclusion, the smart integration of technology in agricultural science research and experimental development is essential for creating a sustainable and efficient food system. It has the potential to increase productivity, reduce waste, and ensure food security for a growing global population. As we continue to innovate and implement these technologies, it is crucial to ensure that they are accessible and beneficial to all stakeholders in the agricultural sector. The future of farming is intelligent, digital, and interconnected, promising a more resilient and prosperous agricultural landscape. 。
纳米对人体健康的作用作文200字
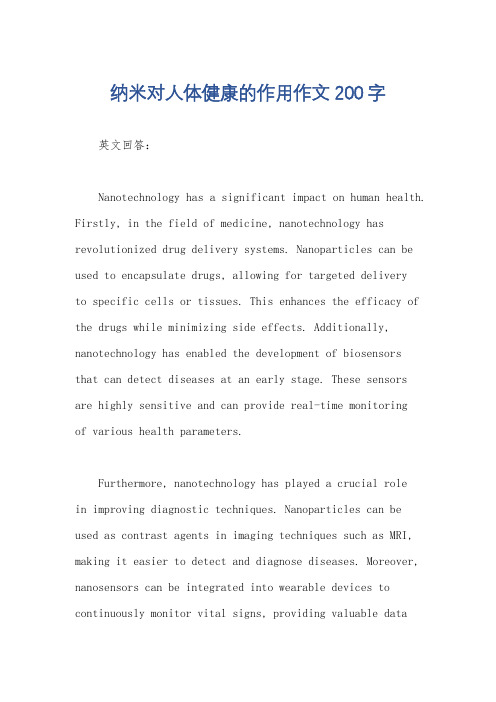
纳米对人体健康的作用作文200字英文回答:Nanotechnology has a significant impact on human health. Firstly, in the field of medicine, nanotechnology has revolutionized drug delivery systems. Nanoparticles can be used to encapsulate drugs, allowing for targeted deliveryto specific cells or tissues. This enhances the efficacy of the drugs while minimizing side effects. Additionally, nanotechnology has enabled the development of biosensorsthat can detect diseases at an early stage. These sensors are highly sensitive and can provide real-time monitoringof various health parameters.Furthermore, nanotechnology has played a crucial rolein improving diagnostic techniques. Nanoparticles can be used as contrast agents in imaging techniques such as MRI, making it easier to detect and diagnose diseases. Moreover, nanosensors can be integrated into wearable devices to continuously monitor vital signs, providing valuable datafor early detection and prevention of health issues.On the other hand, it is important to consider the potential risks associated with nanotechnology. Due totheir small size, nanoparticles can easily enter the human body through inhalation, ingestion, or skin contact. Some studies have shown that certain nanoparticles may havetoxic effects on cells and organs. Therefore, it is crucial to conduct thorough research on the safety and toxicity of nanomaterials before their widespread use in consumer products and medical applications.中文回答:纳米技术对人体健康有着重要的影响。
- 1、下载文档前请自行甄别文档内容的完整性,平台不提供额外的编辑、内容补充、找答案等附加服务。
- 2、"仅部分预览"的文档,不可在线预览部分如存在完整性等问题,可反馈申请退款(可完整预览的文档不适用该条件!)。
- 3、如文档侵犯您的权益,请联系客服反馈,我们会尽快为您处理(人工客服工作时间:9:00-18:30)。
Abstract—The primary goals of ultrasound molecular imag-ing are the detection and imaging of ultrasound contrast agents (microbubbles), which are bound to specific vascular surface receptors. Imaging methods that can sensitively and selectively detect and distinguish bound microbubbles from freely circu-lating microbubbles (free microbubbles) and surrounding tis-sue are critically important for the practical application of ultrasound contrast molecular imaging. Microbubbles excited by low-frequency acoustic pulses emit wide-band echoes with a bandwidth extending beyond 20 MHz; we refer to this tech-nique as transmission at a low frequency and reception at a high frequency (TLRH). Using this wideband, transient echo, we have developed and implemented a targeted imaging technique incorporating a multifrequency colinear array and the Siemens Antares imaging system. The multifrequency colinear array integrates a center 5.4-MHz array, used to receive echoes and produce radiation force, and 2 outer 1.5-MHz arrays used to transmit low-frequency incident pulses. The targeted imaging technique makes use of an acoustic radiation force subsequence to enhance accumulation and a TLRH imaging subsequence to detect bound microbubbles. The radiofrequency (RF) data obtained from the TLRH imaging subsequence are processed to separate echo signatures between tissue, free microbubbles, and bound microbubbles. By imaging biotin-coated microbub-bles targeted to avidin-coated cellulose tubes, we demonstrate that the proposed method has a high contrast-to-tissue ratio (up to 34 dB) and a high sensitivity to bound microbubbles (with the ratio of echoes from bound microbubbles versus free microbubbles extending up to 23 dB). The effects of the imag-ing pulse acoustic pressure, the radiation force subsequence, and the use of various slow-time filters on the targeted imag-ing quality are studied. The TLRH targeted imaging method is demonstrated in this study to provide sensitive and selective detection of bound microbubbles for ultrasound molecularly targeted imaging.I. IntroductionU ltrasound molecular imaging requires several steps: coating microbubble contrast agents with ligands de-signed to bind to receptors on the vascular endothelium; injecting these agents intravenously; and creating an ul-trasound image of their circulation and accumulation [1], [2]. recent studies have shown that targeted ultrasound imaging is a viable tool for the detection and diagnosis of thrombus [3], [4], inflammation [5]–[7], and cancer [8]–[11]. For example, functionalized lipid-based microbubbles tar-geting intercellular adhesion molecule-1 (IcaM-1) have been recently used to detect the overexpression of IcaM-1 during acute cardiac rejection [12].In targeted ultrasound imaging, both the imaging tech-nique and the imaging probe play crucial roles. The tar-geting contrast agent must have high specificity and ef-ficiency, while the imaging technique must be capable of differentiating the echoes of targeted bubbles from those of free microbubbles. low targeting efficiency is a major challenge in the clinical application of ultrasound targeted imaging. In some cases, the density of bound microbubbles retained in the vasculature at a target site has been too low to provide a detectable acoustic signal [13].a further challenge is the differentiation of echoes from bound microbubbles in the presence of free microbubbles [14]. strong background echoes from free microbubbles and tissue obscure echoes from bound microbubbles in conventional multiple-pulse ultrasound contrast imaging, such as pulse inversion (PI) imaging, and thus the poten-tial sensitivity of molecular imaging is lost. lindner et al. [6] proposed an imaging method that acquires echoes from bound microbubbles after the clearance of free mi-crobubbles; however, this method generally incurs a delay of 10 min. To increase the targeting efficiency and imaging specificity, in this paper, we propose the combined use of acoustic radiation force and high contrast-to-tissue ratio (cTr) imaging methods.acoustic radiation force displaces microbubbles to a blood vessel wall, increasing adhesion, particularly within veins and venules [13], [15]–[19]. In our previous study, we demonstrated that the targeting efficacy of microbubbles increases as a result of acoustic radiation force: the num-ber of biotinylated microbubbles bound to a synthetic ves-sel coated with avidin increased as much as 20-fold and the adhesion of microbubbles targeted to αvβ3 expressed on human umbilical vein endothelial cells increased 27-fold within a mimetic vessel when radiation force was ap-plied [17].Microbubbles are unique nonlinear oscillators in an ultrasound acoustic field, producing wideband echoes [20]–[22]. The oscillation-induced nonlinear acoustic back-scatter provides an opportunity to distinguish tissue and microbubble echoes based on their center frequency or re-sponse to the phase of the transmitted pulse [23], [24]. Un-der suitable conditions, an acoustically driven microbub-ble will emit an acoustic transient response [25]–[27]. our recent study found that strong wideband high frequency (5–40 MHz) components were recorded when microbubbles were insonified by 2.25-MHz ultrasound with pressures ofA Sensitive TLRH Targeted Imaging Technique for Ultrasonic Molecular ImagingXiaowen Hu, Hairong Zheng, Member, IEEE, dustin E. Kruse, Patrick sutcliffe, Member, IEEE, douglas n. stephens, Member, IEEE, and Katherine W. Ferrara, Fellow, IEEEManuscript received March 16, 2009; accepted november 13, 2009.This work is supported by nIH ca 103828, ca 112356, and ca 76062.X. Hu, H. Zheng, d. E. Kruse, d. n. stephens, and K. W. Ferrara arewith the department of biomedical Engineering, University of califor-nia, davis, ca (e-mail: kwferrara@).P. sutcliffe is with siemens Medical solutions, Mountain View, ca.digital object Identifier 10.1109/TUFFc.2010.14110885–3010/$25.00 © 2010 IEEE350 to 800 kPa [20]. The high-frequency wideband spec-trum produced by an acoustic transient response can be exploited to improve imaging sensitivity and resolution. Here, we transmit a short pulse with a center frequency near 1.5 MHz, resulting in a broadband ultrasound echo [20]. as compared with transmission and reception of the lower ultrasound frequency, the transmission with a low center frequency and reception with a high center frequen-cy (TlrH) strategy generates a higher resolution image. as compared with transmission and reception of the high-er ultrasound frequency, the TlrH strategy increases the sensitivity to microbubble echoes [14], [20]. The TlrH technique uses a single pulse to image the distribution of microbubbles, whereas PI techniques require at least 2 pulses to cancel the echoes from surrounding tissue. This decrease in the number of necessary transmitted pulses will be crucial for achieving high frame rate 3-d targeted imaging. In addition, TlrH imaging should be insensitive to motion artifacts.In this paper, we investigate the performance of TlrH and new ultrasound arrays for targeted imaging on a com-mercial clinical scanner.II. MethodsA. Frequency-Dependent Transient ResponsePrevious work has been performed by Kruse [20] to pro-duce microbubble transient responses, using the 2.25-MHz transducer listed in Table I. In our work presented here, we optimized the frequency of the imaging pulse for gen-erating the TlrH transient response from microbubbles. Experiments were conducted with various center frequen-cies as listed in Table I, using the experimental system as described by Kruse [20].B. Transducer Array and Clinical Ultrasound System The multifrequency colinear array transducer was inte-grated with the siemens antares system (siemens Medical solutions, Mountain View, ca) for high-resolution TlrH imaging. a “side by side” transducer array was designed to achieve the large difference between the low transmitting and high receiving frequencies used in the TlrH imaging strategy; the center high-frequency array is surrounded on each side by 2 low-frequency arrays [28], [29] (Fig. 1). Each of the 2 outer arrays has 64 elements designed to operate (in transmit-only for TlrH mode) at a center frequency of 1.5 MHz with a −6-db fractional bandwidthof ~50%. The 2 arrays are wired in parallel so that corre-sponding elements of each array are pulsed together. The inner array is comprised of 128 elements designed to oper-ate (in either receive-only in TlrH mode, or otherwise in transmit-receive imaging mode) at 5.4 MHz with a −6-db fractional bandwidth of 73%.Wideband transmission and wideband reception are both essential. based on our previous studies, tissue echoes include frequencies up to the third harmonic; therefore, the center frequency of the receiving transducer array should be higher than the third harmonic, which is 4.5 MHz based on our transmission frequency. With a higher receiving frequency, the separation of bubble echoes and tissue echoes improves. In addition, the lateral and axial resolution will also benefit from the higher re-ceiving frequency. In general, a higher receiving frequency requires more elements in the transducer array and leads to higher cost. Further, the center array is intended to cover the resonance frequency of smaller encapsulated mi-crobubbles and therefore increasing the frequency further is undesirable.Fig. 1. co-linear array transducer face with 3 individual arrays delin-eated: the center high-frequency (5.4 MHz) array is surrounded on each side by low-frequency arrays (1.5 MHz).calibration of the probe was performed with a needle hydrophone (model HnZ-0400, onda corporation, sunny-vale, ca) and an oscilloscope (model 9310 aM, lecroy, chestnut ridge, ny).C. In Vitro Targeted Imaging Flow PhantomWe designed a flow phantom (Fig. 2) consisting of 200-μm-diameter vessel-mimicking cellulose tubes (spec-trum laboratories, rancho dominguez, ca) placed at the focus of the transducer (30–35 mm) and adjacent to tissue-mimicking material. The tissue mimicking material contains 12-μm silicon carbide particles as scatterers with an attenuation of 0.3 db/cm/MHz [30].The microbubbles used in this study are biotin-coated lipid-shelled microbubbles encapsulating a high molecular weight gas core of octafluoropropane [31]. The microbub-ble radius was measured using an accusizer (model 770a, Particle sizing system, santa barbara, ca), with a typi-cal concentration of 109 to 1010 microbubbles per milliliter yielding a mean radius of 0.9 μm.The diluted biotin-targeted microbubbles (104 mi-crobubbles/ml) were pumped by a syringe pump (model PHd 2000, Harvard apparatus, Holliston, Ma) through an avidin coated cellulose tube. The mean flow rate was 5 mm/s, which corresponded to a wall shear stress of 0.2 Pa, a value in the physiologically relevant range for microvessels such as arterioles and venules [32]. The tube was held vertically to eliminate the retention of microbub-bles at the top of the tube due to floatation (Fig. 2). The tissue mimicking material, the tube, and the transducer were immersed in a water bath for acoustic coupling.To ensure that each pulse insonified a new volume of microbubbles, mean travel distance between frames (in-dicating the distance traveled in each frame) should be greater than the −6-db transducer azimuthal beamwidth. The tube is parallel to the surface of the transducer andwithin the imaging (azimuthal-depth) plane. In this study, the mean travel distance between frames was 1 mm, which is greater than the 0.5 mm beamwidth.D. Targeted Imaging TechniqueThe imaging modality makes use of an image-push-im-age (IPI) sequence, illustrated in Fig. 3. a 10-s radiation force subsequence—100-cycle, 5.4-MHz excitations at 200-kPa peak negative pressure (PnP), the PrF is 2.4 kHz—is produced to deflect microbubbles toward the vessel wall and increase microbubble adhesion. Following this, T1r7 (transmit at 1.5 MHz and receive at 7 MHz) images (frame rate is 5 frames/s) are generated by transmitting from the low frequency outer arrays at 1.5 MHz and receiving by the high-frequency center array at 7 MHz, which is near the upper band edge of the array.The echoes received from the TlrH imaging subse-quence are first band-pass filtered, where the band-pass filter is implemented along each scan line within one T1r7 frame (the filter is also called a fast-time filter). The band-pass filter consists of a 6-MHz high-pass filter and a 7-MHz low-pass filter. The low-pass filter is neces-sary to avoid aliasing and the high-pass filter is used to exclude the transmitted passband. as Zhao discussed [14], echoes from free microbubbles can be considered as zero-mean random signals, while the echoes from bound mi-crobubbles have a nonzero mean. Hence, free microbubble echoes are suppressed by applying a low-pass slow-time frame-to-frame filter (Fig. 4). The amplitude threshold, which is set at ~10% of the dynamic range of the slow-time-filtered rF amplitude, is applied to minimize noise. The amplitude threshold value was determined based on the noise level of rF data after the slow-time filter.For the slow-time filter design, we considered 3 types of low-pass filters: an averaging filter, finite impulse response (FIr) filter, and infinite impulse response (IIr) filter. The low cutoff frequencies of the FIr and IIr filters were set as 4% of the doppler frequency shift generated by flow rates of 5 mm/s with a 1.5-MHz incident wave; this corresponds to a low-pass doppler cutoff of 0.2 mm/s or 0.03 Hz. The low cutoff frequency of the averaging filter decreases with increasing filter order. When the order of the averaging filter was 2, the low cutoff frequency was approximately 6 Hz (−20 db); when the order was 17, the low cutoff frequency was approximately 0.67 Hz (−20 db). The pri-mary filters used in this study are listed in Table II.307hu et al.: targeted imaging technique for ultrasonic molecular imagingFig. 2. an illustration of the targeted imaging phantom.Fig. 3. Image-push-image sequence consists of a radiation force subse-quence and a TlrH imaging subsequence.Fig. 5 compares the (a) magnitude and (b) phase re-sponse of 7th-order IIr, FIr, and averaging filter togeth-er. The phase response of the FIr and averaging filters is linear for frequency components below 0.03 Hz; the slope is ~−4.03 rad/Hz. The elliptical low-pass filter has a non-linear phase response near its cutoff frequency. However, for frequency components below 0.03 Hz, the phase re-sponse is approximately linear (r2 = 0.99).The initialization scheme for the IIr filter is critical to the slow-time filter design. In this study, we varied the number of sampling frames from which we calculated the mean values to perform the step initialization [33], and then compared their performance. The number of sam-pling frames was varied from 1 to 30, which was the total number of sampling frames. The initialization of the IIr filter was implemented by the Matlab (MathWorks Inc., natick, Ma) routine “filter.”The PnP of the TlrH imaging pulse was varied within the range of 60 to 330 kPa to study the effect on targeted image quality. To evaluate the effect of the radiation force sequence on targeted microbubble binding, targeted im-ages with and without radiation force were investigated. To study of the quality of T1r7 and T7r7 images, the contrast-to-tissue ratio (cTr*, the star is used here308IEEE TransacTIons on UlTrasonIcs, FErroElEcTrIcs, and FrEqUEncy conTrol, vol. 57, no. 2, FEbrUary2010Fig. 4. descriptive graph of the use of phantom regions, fast-time band-pass filter, and slow-time low-pass filter to separate the bound microbubble echoes from those of tissue and free microbubbles. Fast-time filter and slow-time filter are applied to the radiofrequency (rF) data from the T1r7 imaging subsequence. The fast-time band-pass filter facilitates the discrimination of microbubble echoes from those of tissue; the slow-time filter is designed to differentiate bound and free microbubble echoes.to differentiate the non-targeted definition from the cTr definition in targeted images) is defined asCTR bubblestissue ***log ,=æèçççöø÷÷÷÷10I I (1)where I tissue *is the mean signal intensity in tissue based on T1r7 data; I bubbles *is the mean signal intensity of all mi-crobubbles in the tube based on T1r7 data.To study the quality of targeted images in the phantom experiments, the contrast-to-tissue ratio (cTr) and the bound-to-free microbubble ratio (bFr) are defined. The cTr is defined to quantify the relative signal intensity of bound microbubbles and tissue for T1r7 targeted imag-ing; the bFr is defined to quantify the relative signal in-tensity of bound and free microbubbles for T1r7 targeted imaging. cTr and bFr are calculated byCTR BFR bound tissue bound free =æèçççöø÷÷÷=æèççç1010log ,log I I öø÷÷÷, (2)where using the T1r7 targeted imaging data, I tissue is themean signal intensity in tissue; I free is the mean signal intensity of free microbubbles; I bound is the mean signal intensity of bound microbubbles. In each case, an opera-tor-designed region of interest is used to differentiate re-gions of bound plus free microbubbles, bound or free mi-crobubbles and tissue to study these parameters.The rF data were processed off-line in Matlab.III. resultsA. Frequency-Dependent Transient ResponseThe bandwidth of the transient response increases with decreasing transmitted center frequency (Fig. 6). because we plan to combine thermal therapy and targeted imag-ing together in the future, 1.5 MHz was chosen for the center frequency of the transmitted imaging pulse in the implementation of our combined imaging and therapy ar-ray. In this array, the 5.4-MHz center array receives the microbubble echoes and applies radiation force.B. Linear and TLRH ImageTo compare T1r7 and T7r7 directly for tissue echo re-jection, images of the flow phantom generated using T7r7 (cTr* of 9 db) and T1r7 (cTr* of 28 db) are shown (Fig. 7). The cTr* is then compared as a function of the transmitted PnP of the imaging pulse (Fig. 8), where T1r7 generated a higher cTr* image than T7r7 with an imaging pulse PnP ranging from 60 kPa to 330 kPa. The cTr* of T1r7 and T7r7 peaked at 34 and 10 db, respectively. Thus, increasing incident acoustic pressure increases the ability to differentiate microbubbles from surrounding tissue.C. Performance of Slow-Time Filter The number of sampling frames used to obtain step ini-tialization values affects the performance of the IIr slow-time filter. For example, using the 7th-order IIr filter, a2.5-db increase in cTr and a 5.3-db increase in bFr areobserved when the data on which the initialization value isbased are averaged over 30 sampling frames, as compared with a single sample frame (Fig. 9).Fig. 10 provides an example of targeted images ob-tained by applying a 7th-order averaging filter, FIr filter, and IIr filter to the T1r7 images. The slow-time filter should suppress the echoes from free microbubbles, while retaining the echoes from bound microbubbles.all 3 filters generated similar signal intensity in theregion where microbubbles were bound. However, the averaging filter and FIr filter generated stronger signal309hu et al.: targeted imaging technique for ultrasonic molecular imaging Fig. 5. comparison of the (a) magnitude responses and (b) phase re-sponse of 7th-order IIr, FIr and averaging filter. The FIr and averaging filters have a linear phase response. The phase response of the IIr is ap-proximately linear in the critical frequency range of 0 to 0.03 Hz.intensity in the free microbubble and tube wall region compared with that produced by the IIr filter.The cTr and bFr of images corresponding to the averaging filter, FIr filter, and IIr filter are shown in Fig. 11. The cTr of the IIr filter is 1 db higher than that of the other 2 filters when the filter order is 7. The bFr of the IIr filter is 2.6 db higher than that of the other 2 filters when the filter order is 7.D. Effect of Radiation Force and Imaging Pulse PNP For imaging pulses with PnP from 60 to 330 kPa, the application of radiation force increases the bFr by 7 to 15 db as compared with targeted images obtained with-out radiation force (Fig. 12). When no radiation force subsequence is applied, the increase in bFr with imaging pulse PnP is the result of increased radiation force effect produced by the 1.5-MHz T1r7 imaging pulses. However, the 1.5-MHz transmitting pulses used for T1r7 imaging are less efficient than the 5.4-MHz pulses in producing radiation force [34]. When the radiation force subsequence is applied, as the transmitted PnP of the imaging pulse increases, the bFr also increases, reaching a maximum value (highest sensitivity) of 23 db at an imaging pulse PnP of 250 kPa; however, a further increase in the imag-ing pulse PnP, up to 330 kPa, results in a 3-db decrease in bFr.Fig. 13 provides a series of TlrH targeted images in color obtained as the PnP of the imaging pulse increases from 60 to 330 kPa, using a 7th-order elliptic IIr filter as the slow-time filter and with a constant dynamic range of 40 db. avidin was coated only on the downstream sec-tion of the tube, which was identically coated and placed in each study (as indicated in the cartoon). bound mi-crobubbles, as detected by the algorithms summarized in Fig. 4, are colored in orange and overlaid on the grayscale images. The resulting TlrH targeted images indicate that bound microbubbles were adherent only in the avi-310IEEE TransacTIons on UlTrasonIcs, FErroElEcTrIcs, and FrEqUEncy conTrol , vol. 57, no. 2, FEbrUary2010Fig. 6. The power spectra of transient responses generated by (a) 1 MHz, (b) 2.25 MHz, and (c) 3.2 MHz transmitted pulses. The relative mean spectral power at 15 MHz with varied PnP is plotted in (d).din-coated area; see Figs. 13(b)–(f). bound microbubbles are not detected in the upstream section, which lacks the avidin coating. because acoustic radiation force deflects bubbles from the upper tube wall to the lower tube wall, targeted microbubbles accumulate on the bottom wall, as shown in the TlrH targeted images. The region of bound microbubbles coincides with the location of the avidin coating; although the echogenicity of the coated wall is unchanged in the absence of microbubbles.IV. discussionThe TlrH targeted imaging method, as demonstrat-ed on a clinical ultrasound system, is useful in detect-ing microbubbles and distinguishing bound and free mi-crobubbles. The method in this study should be practical for detecting and imaging ultrasound microbubbles that bind to specific vascular surface receptors. based on the transient response of microbubbles, the proposed method takes advantage of TlrH imaging for microbubble detec-tion, enhancing the rejection of tissue echoes and asso-ciated harmonics. other advantages demonstrated previ-ously include higher sensitivity and penetration depth and better axial resolution than THrH [20].as shown in Fig. 6, the transient response of microbub-bles is highly dependent on the transmitted frequency and PnP of the imaging pulse. In general, lower frequency and higher PnP excitation pulses generate a greater transient response. For example, the mean received power from 1-MHz-driven microbubbles within a frequency range of 6 to 8 MHz increased 10 db as the imaging pulse PnP was increased from 228 kPa to 423 kPa. Increasing the imag-ing pulse PnP increases microbubble destruction; how-ever, bound microbubbles tolerate a greater PnP. Here, the range between 228 to 423 kPa was useful for targeted imaging.The use of lower transmitted frequencies can minimize the interference between the second or third harmon-ics generated by the surrounding tissue and the specific transient response of microbubbles. With our system and a transmitted PnP of 423 kPa, the –6-db transient re-sponse of microbubbles extended to 20 MHz and 15 MHz by transmitting with a 1- and 2.25-MHz center frequen-cies, respectively; see Figs. 6(a)–(b). The third harmonic component produced by the surrounding tissue with these transmitted frequencies is 3 MHz and 6.75 MHz, respec-tively. This implies a broadband receiver can separate311hu et al.: targeted imaging technique for ultrasonic molecular imaging Fig. 7. (a) T7r7 and (b) T1r7 images of control (nontargeted) mi-crobubbles flowing through the flow phantom with an imaging pulse PnP of 250 kPa. The displayed grayscale ranges from the noise floor (~equal in each case) to the peak post-filtered amplitude and the result-ing dynamic range (dr) is ~70 db for T7r7 and ~40 db for T1r7.Fig. 8. comparison of the cTr* obtained from T7r7 and T1r7 imaging with a varied imaging pulse PnP from 60 to 330 kPa.harmonic tissue and bubble echoes. Increasing the center frequency of the transmitted pulse degrades this separa-tion, as we have illustrated in Fig. 6(c) for the 3.2-MHz transmission case.a 7th-order elliptic IIr filter was shown to have the best performance in the detection of bound microbubbles, as compared with the averaging and FIr filter. To ob-serve the effect of the slow-time filter’s filter order, we compared the performance of targeted imaging with filter orders varied from 5 to 17. The cTr and bFr values derived with different filters depend on the stop-band cut-off frequency and transition bandwidth. among the FIr,averaging, and elliptical IIr filter, the elliptical IIr filter achieved the required signal suppression with the smallest filter order. The differences in cTr and bFr between the 7th and 17th order IIr filter are minimal, less than 1 db. longer slow-time filters require more frames to obtain the initialization value and filter, which can increase motion artifacts in the slow-time direction, in turn affecting the accuracy of the filter initialization. In our study, the frame rate was set to 5 frames/s, therefore the total acquisition time for the 7th order filter was ~1.2 s. low-frequency artifacts produced by respiration and other physiological noise sources can be minimized by applying 2-d motion correction. The sum absolute difference (sad) algorithm has been shown to be effective in minimizing motion ar-tifacts in ultrasound imaging and shows promise for tar-geted imaging as well (data not shown). In addition, the radiation force subsequence can induce immediate mi-crobubble adhesion and enhance targeted imaging sensi-tivity (Fig. 12).The slow-time low-pass filter and radiation force based targeted imaging can be integrated with other ultrasound contrast imaging techniques such as contrast pulse se-quence (cPs) and PI.Initial experiments indicated optimal results (highest signal to noise) when the radiation force sequence PnP was 200 kPa, which agrees with our previous in vivo stud-ies [35]. It is likely that some microbubble destruction occurs.312IEEE TransacTIons on UlTrasonIcs, FErroElEcTrIcs, and FrEqUEncy conTrol , vol. 57, no. 2, FEbrUary2010Fig. 9. comparison of the performance (cTr and bFr) of a 7th-order IIr filter using a step initialization value with a mean value estimated by the average value over 1 to 30 sampling frames. The PnP of the imagingpulse is 250 kPa.Fig. 10. Targeted images generated by the (a) FIr filter, (b) averaging filter, and (c) IIr filter are compared. The greatest differences among them are the signal intensity in the free microbubble region circled by the solid ellipse and the tube wall region circled by the dashed ellipse. The PnP of the imaging pulse is 250 kPa.。