Fast Cracking during Fatigue Crack Propagation in Metals
疲劳裂纹扩展速率的实验数据处理

A (1-R)Kc
da/dN B
(1-R)Kc
da/dN C
(1-R)Kc
KthCF D K
(1-R)K1scc D K
(1-R)K1scc D K
A类 ; B类:Kmax<K1scc, (DK)thCF<<DKth 主要是疲劳过程; 腐蚀使(da/dN)CF Kmax>K1scc, 腐蚀 使da/dN)CF。 普遍加快,如铝 合金在淡水中。 马氏体镍在干氢中.
DKth Mpa.m1/2
8 7 6 5 4 3 2 1
低碳钢 低合金钢 不锈钢 A517-F
9301 A508C A533B
R 不同钢材的R-DKth 关系 lgda/dN
R=0.8 0 -1
0 .2
.4
.6
.8 1.0
R<0的情况:负应力存在, 对da/dN三区域的影响不同。 情况比R>0时复杂得多。
lgda/dN
8.4 疲劳裂纹扩展速率试验
0
a (mm)
D =const. R=0
Dai DK 曲线 目的:测定材料的 da/dNa DNi
一、试验原理:
Paris公式: 实验 a =a 0 R=0 D
N
lg(DK)
da/dN=C(DK)m (DK)i=f (D,ai,)
记录ai、Ni
ai=(ai+1+ai)/2
12
In general, at low frequencies, crack growth rate 在空气中,一般观察不到波形对疲劳裂纹扩展速 increase as more time is allowed for environmental 率的影响。但在腐蚀环境中,若载荷循环的拉伸 attack during the fatigue process. 部分作用慢, da/dN 一般较高。
Fatigue Crack Growth Analysis of Fiber
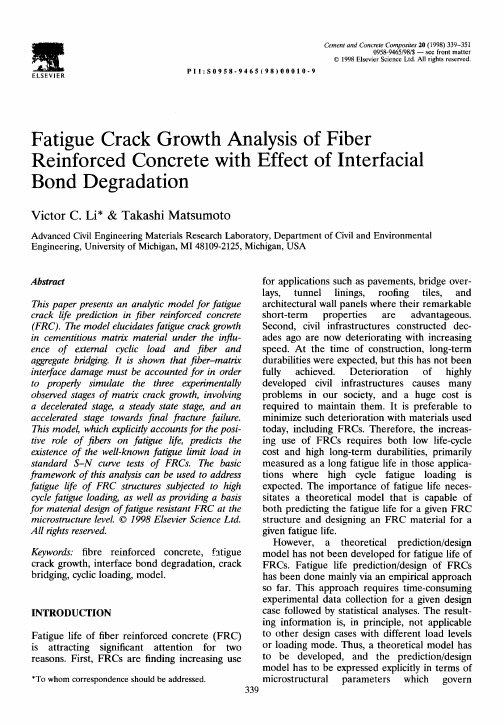
number of microscopic changes during fatigue loading, fatigue crack growth can be considered the damage most responsible for fatigue life of FRCs and, therefore, must be focused on. FRCs fail in fatigue when a fracture propagates unstably subsequent to stable crack growth under fatigue loading.4*5 Thus, fatigue life of FRCs is controlled by fatigue crack growth behavior. Fatigue crack growth in FRCs is affected by three main factors: matrix fatigue crack growth law specific to a matrix, crack bridging by fibers, and fatigue damage in the fiber-matrix interface and/or bridging fibers. These three factors are discussed in the following sections. First, matrix fatigue crack growth is quantified with the Paris law, which relates the crack growth rate to the intensity factor amplitude. crack-tip-stress Second, a cyclic constitutive law between the crack bridging stress amplitude and the crack opening displacement amplitude due to fibers and aggregates is described. With the cyclic constitutive law, the crack-tip-stress intensity factor amplitude, which is reduced by crack bridging, can be evaluated. Finally, the interfacial frictional bond degradation under cyclic sliding is quantified assuming that the bond degradation is governed by the accumulated crack opening displacement change. Fatigue damage on matrix: fatigue crack growth Fatigue crack growth has been observed to obey a Paris law type equation for metals, ceramics, and concrete.6-‘9 The Paris law gives the relation between the crack growth rate and the intensity factor amplitude, crack-tip-stress namely
Assessment of Fatigue Cracks in Rails

Social and Behavioral Sciences Procedia - Social and Behavioral Sciences 00 (2011) 000–000 /locate/procediaConference Title (Transport Research Arena – Europe 2012)Assessment of Fatigue Cracks in Rails George Kotsikos *a Marzio Grasso ba School of Mechanical & Systems Engineering, Stephenson Building, Newcastle University, Newcastle upon Tyne NE17RU, UKb University Federico II, P.le V. Tecchio. 80, 80125 Naples, ItalyAbstractThe fatigue behaviour of cracks at the foot region of a rail subjected to bending load has been investigated. Depending on the position of initiation of a small semi-elliptical surface crack in a rail, the crack front changes shape during propagation to failure due to the large variation of the stress intensity factor from point to point round the crack front due to differences in the local stress field as each point round the crack front lies at different distances from the neutral axis of the rail. This condition implies a variable crack growth rate that transforms the crack front shape during fatigue crack propagation. SIF values have been estimated by means of both the finite element method and analytical solutions derived for a semi-elliptical crack in a finite rectangular cross-section beam. The SIF value predictions obtained with the two methods show good agreement suggesting that the analytical solutions can be used for a rapid assessment of the severity of a flaw in a rail. A predictive model for crack growth has been derived for an initial small crack at an initiation point at the foot/web corner of a rail tested under four point bending fatigue in the laboratory, showing a reasonably good prediction of both the shape and size of the crack at failure when compared with experiment.© 2011 Published by Elsevier Ltd. Selection and peer-review under responsibility of TRA 2012Keywords: fatigue, rail crack, fracture mechanics, FEA1. IntroductionThe fracture of a rail as a result of the development of fatigue cracking is considered as a serious eventin the rail industry as it is likely to result in vehicle derailment with a high probability of loss of life. Cracks in rails have been appearing on the head of the rail as a result of mechanical damage due to wheel /rail contact stresses, or the foot of the rail emanating from corrosion pits. More recently, since the method of joining rails by fishplates was abandoned in favour of continuous welded rail, another source of*Corresponding author. Tel.: +44 191 2225889; fax: +44 191 2225821.E-mail address : george.kotsikos@.2Author name / Procedia - Social and Behavioral Sciences 00 (2011) 000–000cracking was introduced arising from various weld defects (e.g. porosity, lack of fusion, shrinkage stresses etc.). Infrastructure managers are devoting a large amount of effort to ensure that the integrity of the rail track network is preserved by conducting regular inspections involving up to date NDE techniques. Nevertheless, the development of cracks in rails is unavoidable. The rail damage prediction tools (such as VAMPIRE) currently employed by the industry are based on empirical relations based on the cumulative damage sustained by the axle weight and volume of traffic passing over a rail (J. Evans, 2003). These models do not take into account the development or presence of flaws. It can be stated that decisions on replacement of a rail are not made on the basis of a fracture mechanics based flaw severity assessment (a damage tolerance approach).This work has undertaken the study of fatigue cracks in rails using FEA modeling and analytical solutions to provide a tool to rail NDE inspectors for the quick assessment of the severity of surface breaking and embedded cracks in rails. The work does not investigate crack initiation and propagation phenomena on the heads of rails, but concentrates mainly on cracks initiating and propagating from bending loads in the foot of the rail as a result of passing trains.1.BackgroundThe propagation of a crack is driven by the stress field that develops ahead of the crack tip. In fracture mechanics, the stress and strain fields can be characterized by parameters such as the stress intensity factor, K, under elastic conditions, or the J-integral or crack tip opening displacement(CTOD) under conditions of extended plasticity. Such parameters describe the mechanics of the crack in terms that include the applied load and the length of the crack. The resistance of a material to fast fracture is given by the fracture toughness. Under small scale yielding conditions, to predict crack propagation life and fracture strength, accurate stress-intensity factor solutions are needed both for initial, intermediate and final crack configurations. But, because of the complexities of such problems, exact solutions are not available. Instead, investigators have had to use approximate analytical methods, experimental methods, or engineering estimates to obtain the stress-intensity factors. Closed form solutions for stress intensity factors of elliptical cracks in infinite bodies have been derived by several authors (Green-1950, Irwin-1962, Kassir-1966, Kobayashi-1976, Newman-1979). For finite bodies, all solutions have required approximate analytical methods with the use of finite element analysis (FEA) or boundary element analysis (BEA) methods (Nishioka-1983, Raju-1979, Shah-1971, Smith -1967, Tracey -1973, Vijakumar-1981, Pan -1999).In this paper, a numerical approach that takes into account not only the finite geometry of the defected component but also the real elastoplastic behaviour of the component material has been applied. The implemented numerical model has been validated by means of full scale crack propagation test on defected rails.2.Analytical solutionThe stress intensity factor expression used in this work is given by: K = S F b√(πa/Q)where, S, is the remote applied stress (tensile or bending), a, is the maximum crack depth, Q, is the shape factor for an ellipse given by the square of the complete elliptic integral of the second kind, and F b, is the boundary correction factor which accounts for the influence of the various boundaries. The definition of F b is important and various authors have provided numerous expressions based on FEA parametricAuthor name / Procedia - Social and Behavioral Sciences 00 (2011) 000–000 3 studies. As a starting point the expressions derived by Newman & Raju have been used in this work. A detailed description of these expressions can be found in reference Raju & Newman (1979).3.ExperimentalMechanical property tests were carried out according to BS EN 10002-1:2001 to obtain the rail material mechanical properties for the analysis. Specimens were obtained from the rail head, web andbase along the longitudinal and transverse direction to the rail. There was little variation in the tensile properties between the different regions of the rail. The average yield strength was determined as 540MPaand the ultimate strength 940MPa with an elongation of approximately 12%.Fracture mechanics tests were conducted according to BS7448 Part 2. DCB specimens were extractedfrom the head, web and base of the rail so that the notch (and crack extension path) would lie in the transverse direction to the rail, which is also the direction cracks grow in service.Figure 1. Position of extraction of tensile and fracture mechanics test samples from a UIC60 rail.The fracture toughness K IC of the material could not be determined because the size of the specimensthat could be extracted from the rail would not yield valid results. The K Q value was determined and wasfound to be approximately 27 MN∙m-3/2 (±2 MN∙m-3/2) at all specimen positions in the rail.Constant load amplitude four point bending fatigue tests were carried out on a section of a rail toassess the accuracy of the numerical and analytical solutions. A UIC60 rail section, the most common railprofile in European railways, was used. The rail support span was 750mm and the loading point span190mm. The maximum applied fatigue load was 582kN with a frequency of 1Hz and an R ratio of 0.3(R=P min/P max). The fatigue tests were alloed to continue until complete fracture of the rail took place.Once a crack had initiated, the test was stopped and the crack heat tinted in order to determine the shapeof the crack front just after initiation and how this changes as the crack front propagates to failure.Figure 2. Flaws on fatigued samples, semicircular crack at rail base (left), elliptical crack at bottom web corner (right)4Author name / Procedia - Social and Behavioral Sciences 00 (2011) 000–0004. FEAThe finite element analysis has been carried out using the ANSYS package. Singular quarter-point elements for the small region around the crack front, where the stress field is K-dominated, have been used. The quarter-point elements allow the modelling of singular displacement fields and thus the evaluation of the stress intensity factor (SIF) with great accuracy and a relatively coarse mesh. Several methods are actually available to obtain the SIF values during the post processing of the finite element solution. Among them, the Displacement Correlation Method (DCM) , applicable only when the material has linear elastic isotropic behaviour, makes use of the well-known relations between the SIF’s values and the displacement field around the crack front. Otherwise, knowing the stress and strain field, the J values along the crack front have to be numerically evaluated and from them the SIF values can be derived.The scientific code FRANC3D version 5.0, developed by Cornell University, was used to generate the mesh around the crack front, which allows for both the preprocessing, i.e. it allows the creation of the starter crack in the model, and the postprocessing, i.e. the calculation of the SIF in all the nodes of the crack front created. Starting from these SIF values, a prediction of crack growth has been attempted using Paris’ law which relates the crack growth rate with the stress intensity factor in the form of the expression:da/dN=C K mIn this work, the parameters C and m were obtained by means of crack propagation tests on DCB specimens, extracted from a rail. The values were: C = 3.3×10-13 and m =2.63Figure 3. Outline of fatigue crack fronts at failure.Figure 3 shows a number of crack fronts of fatigue cracks at failure during fatigue testing. The crack geometries were carefully measured and used for comparisoon with the FEA model and the analytical solutions. Comparative results of the FEA and analytical solution for crack 1 and crack 3 are given in Figure 4. It can be seen that there is a good agreement of the SIF values between FEA and analytical solution for both crack fronts. The FEA shows an increase in the SIF values as the crack front ends approach the surface. This is a result of how the FRANC3D code treats the singularity near the free surface of the material. The singularity is described by 1/r0.5and is approximated by the quarter pointAuthor name / Procedia - Social and Behavioral Sciences 00 (2011) 000–000 5 element. This is true along the crack front but not thenpoint where the crack front intersects the externalsurface and the quarter point element does not describe in a proper manner the singularity because thepower of trhe singularity depends on the angle between the front and the external surface and the Poissonratio.Figure 4. Comparison of SIF values from FEA and analytical solution for web corner Crack 1 (left) and crack 3(right).Also, in the present calculation, the M-integral method was used to evaluate stress intensity factors. Therefore, the stress intensity factor calculated for the surface point was in fact an average value over the element size. The stress intensity factor for the surface point of the surface crack should be considered a reasonable physical approximation of the state of affairs at the surface.Figure 5. Semi-circular crack on base of rail 30mm from the CLThe modelling then examined the development of cracks from the foot of the rail, (Figure 2-left). A comparison of the results is shown in Figure 5. In a similar manner to the web corner cracking, the analytical model also adopted the Newman & Raju solutions for bending stress incorporating a bending correction factor, H j, for the component geometry. For the rail geometry the bending factor overestimatedthe stress level and was not appropriate for prediction of SIFs. A better agreement between the FEAmodels and the analytical solution was achieved by considering the loading on the rail as a variabletensile stress the magnitude of which is a function of the distance from the neutral axis according to the equation s=My/I where, M is the bending moment, I the moment of intertia of the rail and y the distancefrom the neutral axis.6Author name / Procedia - Social and Behavioral Sciences 00 (2011) 000–0005.Crack Propagation predictive modelIt was observed that the crack initially has a semi-circular profile which changes to elliptical as the crack propagates. The crack front extends further towards the foot of the rail and less towards the web. This is reasonable as the stress increases the further away from the neutral axis towards the foot of the rail.Figure 6. Fatigue tested rail showing semicircular crack near initiation site (solid line)and semi-elliptical crack atfailure (dotted line).Since the aforementioned practice does not take into account the material ductility, a special code has been implemented in MATLAB, to correct the crack front shape with the plastic radius and evaluate the effective SIF values along the front. By the theoretical SIF values, K I, and the following expression:r p=( /8)(K I/Y)2where, Y is the material yield stress. The plastic radius values, r p, along the front have been evaluated, and a virtual crack front equivalent to the real one, but embedded in an elastic medium, has been generated. The front so created was imported in FRANC3D to evaluate the effective ΔK and the local increment Δa of the crack front, in order to o btain the new “theoretical” crack front corresponding to a given increment of number of cycles.Figure 7. Crack fronts obtained numerically starting from an initial semicircular flaw with radius equal to 2 mm.Author name / Procedia - Social and Behavioral Sciences 00 (2011) 000–000 7 In Figure 7 a series of crack fronts during fatigue crack propagation to failure obtained from an initialflaw of semicircular shape and 2 mm radius are presented. The initial crack is located near the corner between the foot and web of the rail where the center of the semicircle is positioned on the externalsurface and 27 mm distance from the underside of the rail foot. This simulates the fatigue crack of figure6. A comparison of the crack fronts at failure of Figures 6 and 7 shows not a perfect but a reasonablyclose prediction of the fatigue crack final front. This result also confirms the need of take account of theplastic region around the crack tip when the fatigue crack growth phenomenon in a ductile material has tobe simulated.6.ConclusionsAnalytical solutions based on the Newman & Raju equations to obtain the stress intensity factorsround the crack front of cracks in rails have been derived. These were compared with FEA data using ANSYS incorporating the FRANC3D code. The results show a good agreement if a modification is madeto the Newman & Raju equations whereby the applied stress is treated as a variable tensile stress ratherthan bending. The results were validated via experimental four point fatigue tests in rails.A finite element analysis of the fatigue crack growth near the foot of a rail was carried out by means ofa commercial code integrated with an ad hoc macro written to iteratively evaluate the plastic radius, sincethe rail material is ductile, and the eff ective ΔK value that has to be used to evaluate the local growth ofthe crack front.The good agreement between numerical result and experimental data obtained by fatigue testing of aUIC60 rail segment with the same geometry, made of the same material, having the same defect and subjected to same load used in the numerical simulation, confirms the validity of the adopted modeling procedure and the need of directly introduce the effect of the plastic region at the crack tip when the problem of the crack growth in a ductile material has to be faced.7.ReferencesJ. Evans. “Whole life rail model application and development for RSSB- Dynamic modeling of rolling contact fatigue”, AEA Technology Rail report AEATR-VTI-2003-048 Issue 1, , (2003).Green, A. E. and Sneddon, I. N.: The Distribution of Stress in the Neighbourhood of a Flat EllipticalCrack in an Elastic Solid, Proc. Cambridge Phil. Soc., Vol. 47, 1950, pp. 159-164.Irwin, G. R.: The Crack Extension Force for a Part-Through Crack in a Plate, ASME, Journal of Applied Mechanics, Vol. 29, No. 4, 1962, pp. 651-654.Kassir, M. K. and Sih, G. C., Three-Dimensional Stress Distribution Around an Elliptical Crack Under Arbitrary Loadings, Journal of Applied Mechanics, Vol. 88, 1966, pp. 601-611.Kobayashi, A. S.: Crack-Opening Displacement in a Surface Flawed Plate Subjected to Tension or Plate Bending, Proc. Second Intl_Conf. on Mechanical Behaviour of Materials, ASM, 1976, pp. 1073-1077. Newman, J. C., Jr._ and Raju, I. S.: Analyses of Surface Cracks in Finite Plates under Tension or Bending Loads, NASA TP-1578, Dec. 1979.8Author name / Procedia - Social and Behavioral Sciences 00 (2011) 000–000Nishioka, T.; and Atiuri, S. N.: Analytical Solution for Embedded Elliptical Cracks, and Finite Element-Alternating Method for Elliptical Surface Cracks, Subjected to Arbitrary Loadings, Engineering Fracture Mechanics, Vol. 17, 1983, pp. 247-268.Raju, I. S.; and Newman, J. C., Jr.: Stress-Intensity Factors for a Wide Range of Semi-Elliptical Surface Cracks in Finite-Thickness Plates, Engineering Fracture Mechanics J., Vol. ii, No. 4, 1979, pp. 817-829.Shah, R. C.; and Kobayashi, A. S.: Stress-Intensity Factor for an Elliptical Crack Under Arbitrary Normal Loading, Engineering Fracture Mechanics, Vol. 3, 1971, pp. 71-96.Smith, F. W.; Emery, A. F.; and Kobayashi, A. S.: Stress Intensity Factors for Semi-Circular Cracks, Part 2 - Semi-Infinite Solid, Journal of Applied Mechanics, Vol. 34, No. 4, Trans. ASME, Vol. 89, Series E, Dec. 1967, pp. 953-959.Tracey, D. M.,: 3D Elastic Singularity Element for Evaluation of K Along an Arbitrary Crack Front, Int. J. of Fracture, Vol. 9, 1973, pp. 340-343.Vijayakumar, Ko; and Atluri, S. N.: An Embedded Elliptical Flow in an Infinite Solid, Subject to Arbitrary Crack-Face Tractions, Journal of Applied Mechanics, Vol. 48, 1981, pp. 88-96.Pan, E.; Amadei, B.: Boundary Element analysis of fracture mechanics in anisotropic materials, Engineering analysis with Boundary Elements, Vol 23, (1999), pp. 683-691。
钢桥面板U肋与横隔板不同连接缺口形式受力分析
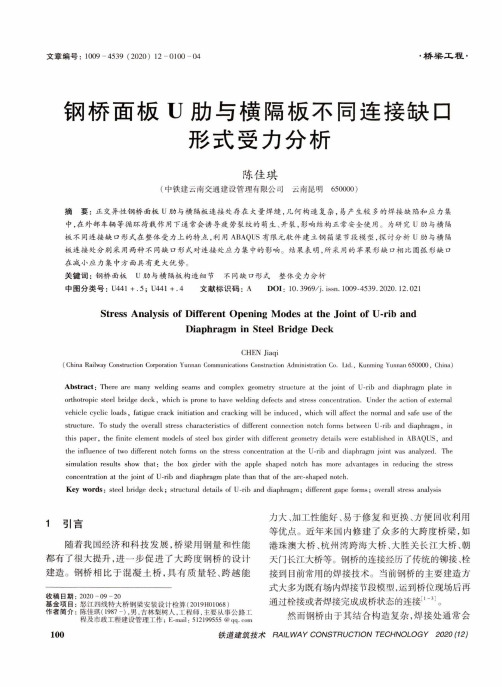
文章编号:1009-4539(2020)12-0100-04•桥梁工程•钢桥面板U肋与横隔板不同连接缺口形式受力分析陈佳琪(中铁建云南交通建设管理有限公司云南昆明65_)摘要:正交异性钢桥面板U肋与横隔板连接处存在大量焊缝,几何构造复杂,易产生较多的焊接缺陷和应力集中,在外部车辆等循环荷栽作用下通常会诱导疲劳裂纹的萌生、开裂,影响结构正常安全使用。
为研究U肋与横隔 板不同连接缺口形式在整体受力上的特点,利用A B A Q U S有限元软件建立钢箱梁节段模型,探讨分析U肋与横隔 板连接处分别采用两种不同缺口形式对连接处应力集中的影响。
结果表明,所采用的苹果形缺口相比圆弧形缺口在减小应力集中方面具有更大优势。
关键词:钢桥面板U肋与横隔板构造细节不同缺口形式整体受力分析中图分类号:U441+.5;U441+.4文献标识码:A D O I:10. 3969/j. issn. 1009-4539.2020. 12.021Stress Analysis of Different Opening Modes at the Joint of U-rib andDiaphragm in Steel Bridge DeckC H E N Jiaqi(China Railway Construction Corporation Yu n n a n Communications Construction Administration Co. Ltd., K unming Yunnan 650000, China)A b stra c t:T h e r e are m a n y welding s e a m s a n d c o m p l e x geometry structure at the joint of U-rib a n d diaph r a g m plate inorthotropic steel bridge d e c k,w h i c h is prone to h a v e welding defects a n d stress concentration. U n d e r the action of external vehicle cyclic loads, fatigue crack initiation a n d cracking will be induced, w h i c h will affect the normal a n d safe use of the structure. T o study the overall stress characteristics of different connection notch forms b etween U-rib a n d diaphragm, in this paper, the finite element m o d e l s of steel box girder with different geometry details were established in A B A Q U S,and the influence of two different notch forms o n the stress concentration at the U-rib a n d d i a p h r a g m joint w a s analyzed. T h esimulation results s h o w that:the box girder with the apple sh a p e d notch has m o r e advantages in reducing the stress concentration at the joint of U-rib a n d d i a p h r a g m plate than that of the arc-shaped notch.Key w o rd s:steel bridge d e c k;structural details of U-rib a n d d i a p h r a g m;different gape forms; overall stress analysis1引言随着我国经济和科技发展,桥梁用钢量和性能 都有了很大提升,进一步促进了大跨度钢桥的设计 建造。
空客A380疲劳裂缝交通运输管理知识分析(英文版)
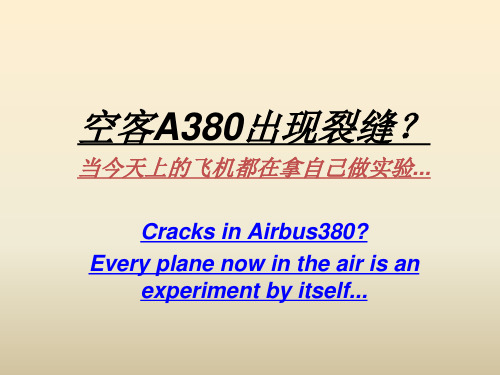
At first, Airbus officials said that it would have to sell about 250 aircraft to recoup
its costs. But according to the Latest estimates by aviation experts, the company will have to sell at least 600 units of the world's largest aircraft to make a profit. But with a total of 253 orders on its books so far, Airbus is still a
Tom Williams, the executive vice president of programs at Toulouse-based Airbus, and his creative engineers were told to subject the A380 to a radical diet.
…the size of the turbines and wings also increase, which in turn increases weight and fuel consumption.
On the other hand, the European megaliner was also required to be 20 percent more efficient than the competing Boeing 747.
long way from that target. 350 MORE A380 FOR BREAK EVEN POINT, i.e. > US$
航空关键动部件的开裂分析及检测应用
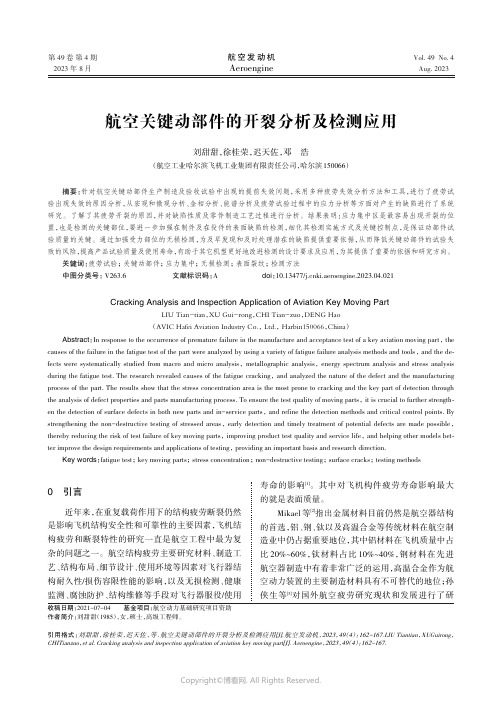
收稿日期:2021-07-04基金项目:航空动力基础研究项目资助作者简介:刘甜甜(1985),女,硕士,高级工程师。
引用格式:刘甜甜,徐桂荣,迟天佐,等.航空关键动部件的开裂分析及检测应用[J].航空发动机,2023,49(4):162-167.LIU Tiantian ,XUGuirong ,CHITianzuo ,et al.Cracking analysis and inspection application of aviation key moving part[J].Aeroengine ,2023,49(4):162-167.第49卷第4期2023年8月Vol.49No.4Aug.2023航空发动机Aeroengine航空关键动部件的开裂分析及检测应用刘甜甜,徐桂荣,迟天佐,邓浩(航空工业哈尔滨飞机工业集团有限责任公司,哈尔滨150066)摘要:针对航空关键动部件生产制造及验收试验中出现的提前失效问题,采用多种疲劳失效分析方法和工具,进行了疲劳试验出现失效的原因分析,从宏观和微观分析、金相分析、能谱分析及疲劳试验过程中的应力分析等方面对产生的缺陷进行了系统研究。
了解了其疲劳开裂的原因,并对缺陷性质及零件制造工艺过程进行分析。
结果表明:应力集中区是最容易出现开裂的位置,也是检测的关键部位,要进一步加强在制件及在役件的表面缺陷的检测,细化其检测实施方式及关键控制点,是保证动部件试验质量的关键。
通过加强受力部位的无损检测,为及早发现和及时处理潜在的缺陷提供重要依据,从而降低关键动部件的试验失败的风险,提高产品试验质量及使用寿命,有助于其它机型更好地改进检测的设计要求及应用,为其提供了重要的依据和研究方向。
关键词:疲劳试验;关键动部件;应力集中;无损检测;表面裂纹;检测方法中图分类号:V263.6文献标识码:Adoi :10.13477/ki.aeroengine.2023.04.021Cracking Analysis and Inspection Application of Aviation Key Moving PartLIU Tian-tian ,XU Gui-rong ,CHI Tian-zuo ,DENG Hao (AVIC Hafei Aviation Industry Co.,Ltd.,Harbin150066,China )Abstract :In response to the occurrence of premature failure in the manufacture and acceptance test of a key aviation moving part ,the causes of the failure in the fatigue test of the part were analyzed by using a variety of fatigue failure analysis methods and tools ,and the de⁃fects were systematically studied from macro and micro analysis ,metallographic analysis ,energy spectrum analysis and stress analysis during the fatigue test.The research revealed causes of the fatigue cracking ,and analyzed the nature of the defect and the manufacturing process of the part.The results show that the stress concentration area is the most prone to cracking and the key part of detection throughthe analysis of defect properties and parts manufacturing process.To ensure the test quality of moving parts ,it is crucial to further strength⁃en the detection of surface defects in both new parts and in-service parts ,and refine the detection methods and critical control points.By strengthening the non-destructive testing of stressed areas ,early detection and timely treatment of potential defects are made possible ,thereby reducing the risk of test failure of key moving parts ,improving product test quality and service life ,and helping other models bet⁃ter improve the design requirements and applications of testing ,providing an important basis and research direction.Key words :fatigue test ;key moving parts ;stress concentration ;non-destructive testing ;surface cracks ;testing methods0引言近年来,在重复载荷作用下的结构疲劳断裂仍然是影响飞机结构安全性和可靠性的主要因素,飞机结构疲劳和断裂特性的研究一直是航空工程中最为复杂的问题之一。
Fatigue crack propagation of 444 stainless steel welded joints in air and in 3%NaCl aqueous solution
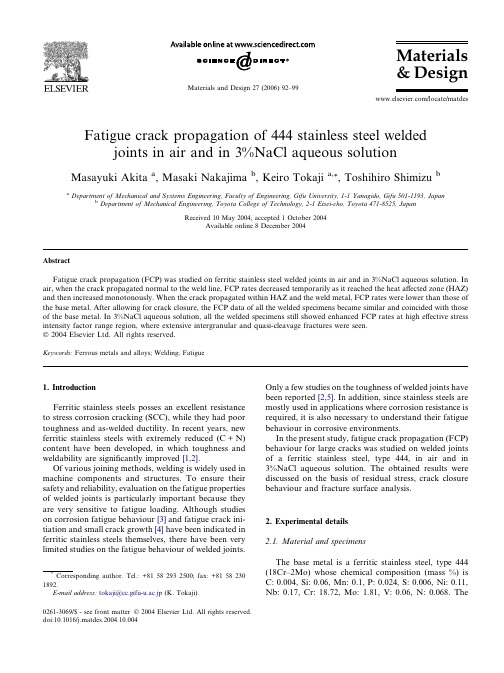
Fatigue crack propagation of 444stainless steel weldedjoints in air and in 3%NaCl aqueous solutionMasayuki Akita a ,Masaki Nakajima b ,Keiro Tokajia,*,Toshihiro ShimizubaDepartment of Mechanical and Systems Engineering,Faculty of Engineering,Gifu University,1-1Yanagido,Gifu 501-1193,JapanbDepartment of Mechanical Engineering,Toyota College of Technology,2-1Eisei-cho,Toyota 471-8525,JapanReceived 10May 2004;accepted 1October 2004Available online 8December 2004AbstractFatigue crack propagation (FCP)was studied on ferritic stainless steel welded joints in air and in 3%NaCl aqueous solution.In air,when the crack propagated normal to the weld line,FCP rates decreased temporarily as it reached the heat affected zone (HAZ)and then increased monotonously.When the crack propagated within HAZ and the weld metal,FCP rates were lower than those of the base metal.After allowing for crack closure,the FCP data of all the welded specimens became similar and coincided with those of the base metal.In 3%NaCl aqueous solution,all the welded specimens still showed enhanced FCP rates at high effective stress intensity factor range region,where extensive intergranular and quasi-cleavage fractures were seen.Ó2004Elsevier Ltd.All rights reserved.Keywords:Ferrous metals and alloys;Welding;Fatigue1.IntroductionFerritic stainless steels posses an excellent resistance to stress corrosion cracking (SCC),while they had poor toughness and as-welded ductility.In recent years,new ferritic stainless steels with extremely reduced (C +N)content have been developed,in which toughness and weldability are significantly improved [1,2].Of various joining methods,welding is widely used in machine components and structures.To ensure their safety and reliability,evaluation on the fatigue properties of welded joints is particularly important because they are very sensitive to fatigue loading.Although studies on corrosion fatigue behaviour [3]and fatigue crack ini-tiation and small crack growth [4]have been indicated in ferritic stainless steels themselves,there have been very limited studies on the fatigue behaviour of welded joints.Only a few studies on the toughness of welded joints have been reported [2,5].In addition,since stainless steels are mostly used in applications where corrosion resistance is required,it is also necessary to understand their fatigue behaviour in corrosive environments.In the present study,fatigue crack propagation (FCP)behaviour for large cracks was studied on welded joints of a ferritic stainless steel,type 444,in air and in 3%NaCl aqueous solution.The obtained results were discussed on the basis of residual stress,crack closure behaviour and fracture surface analysis.2.Experimental details 2.1.Material and specimensThe base metal is a ferritic stainless steel,type 444(18Cr–2Mo)whose chemical composition (mass %)is C:0.004,Si:0.06,Mn:0.1,P:0.024,S:0.006,Ni:0.11,Nb:0.17,Cr:18.72,Mo:1.81,V:0.06,N:0.068.The0261-3069/$-see front matter Ó2004Elsevier Ltd.All rights reserved.doi:10.1016/j.matdes.2004.10.004*Corresponding author.Tel.:+81582932500;fax:+81582301892.E-mail address:tokaji@cc.gifu-u.ac.jp (K.Tokaji)./locate/matdesMaterials and Design 27(2006)92–99Materials &Designmaterial was used in the as-received condition.The mechanical properties for two directions parallel and perpendicular to the rolling direction(L and T direc-tions,respectively)are listed in Table1.The proof stress and tensile strength in the T-direction are slightly higher than those in the L-direction.Fig.1shows the configuration of compact type(CT) specimens used in FCP experiment.As illustrated schemat-ically in Fig.2,three types of welded CT specimens were evaluated:the specimens of which starter notch was intro-duced perpendicular to the weld line(N-specimen,Fig. 2(a)),within the heat affected zone(HAZ)(H-specimen, Fig.2(b)),and within the weld metal(W-specimen,Fig. 2(c)).Since the welding direction was parallel to the T-direc-tion,the FCP direction was the L-direction in the N-speci-mens,while the T-direction in the H and W specimens. 2.2.Welding conditionWelding was performed by the tungsten–inert gaswelding method(TIG)with a root opening of1.6mm, an X-shape groove,the voltage of20V and three weld-ing passes on both sides.Thefiller metal was a309L austenitic stainless steel.The microstructures in the base metal,HAZ and the weld metal are represented in Fig.3.The microstructure of the base metal consists of ferritic grains with the aver-age size of approximately56l m(Fig.3(a)).In HAZ,the microstructure also consists of ferritic grains,but a pro-nounced grain growth takes place,resulting in the aver-age size of123l m(Fig.3(b)).On the other hand,the weld metal has a complicated microstructure:dendrite or acicular microstructure(Fig.3(c)).Hardness was measured using a Vickers hardness tes-ter and the obtained results were162HV,172HV and 194HV for the base metal,HAZ and the weld metal, respectively.2.3.ProceduresFCP tests were performed at a stress ratio,R,of0.05 using a19.6kN capacity electro-hydraulic fatigueTable1Mechanical properties of base metalDirection0.2%Proof stressr0.2(MPa)Tensile strengthr B(MPa)Breaking strength onfinal area r T(MPa)Elongationu(%)Reduction of areaw(%)L29344514803483T31046013523181M.Akita et al./Materials and Design27(2006)92–9993testing machine operating at a frequency of 1Hz.Test environments employed were laboratory air and 3%NaCl aqueous solution.The solution was kept at 30°C and circulated between the corrosion cell attached to the specimen surface and a reserved tank by using a pump.Crack length was monitored with a travelling micro-scope with a resolution of 10l m and crack closure was measured by an unloading elastic compliance method [6]using a strain gauge mounted on the back face of the specimen.After experiment,fracture surfaces were examined in detail using a scanning electron microscope (SEM).3.Results3.1.Residual stress distributionResidual stress in the welded specimens was measured by the X-ray diffraction method.Fig.4shows the distribu-tion of residual stresses normal to the FCP direction in the N-specimens.Residual stresses are approximately from À110to À480MPa at the top surface (the side of the firstpass),while À100to À500MPa at the bottom surface (the opposite side of the second pass).Compressive residual stresses decrease as the distance from the notch root in-creases toward the weld zone.From this measured resid-ual stress distributions,it is believed that large tensile residual stresses would be present in the weld metal.Residual stresses normal to the weld line were also measured in HAZ,which were approximately À300MPa (compression)at the top surface,while 100MPa (tension)at the bottom surface,regardless of the dis-tance from the weld zone.3.2.FCP behaviour of base metalAs described previously,the FCP direction is the L-direction in N-specimens,while the T-direction in H and W specimens,thus FCP behaviour for the base me-tal was evaluated using CT-specimens of both T–L and L–T orientations.Fig.5(a)represents the relationship between FCP rate,d a /d N ,and stress intensity factor range,D K ,for the base metal in air and in 3%NaCl aqueous solution.As can be seen in the figure,the effect of orientation is not seen at high D K region in both environments,but the FCP rates for the L–T orientation are faster than those for the T–L orientation at low D K region.It is worth noting that the FCP behaviour for both orienta-tions is affected by corrosive environment at D K P 15MPa m 1/2,where the FCP rates in 3%NaCl aqueous solution are significantly faster than those in air.When the FCP data are characterized in terms of the effective stress intensity factor,D K eff(Fig.5(b)),the dif-ference in FCP rate between both orientations observed in the d a /d N –D K relationship disappears.This indicates that crack closure played a significant role in FCP behaviour.Based on observation of crack path and frac-ture surface analysis,fracture surface roughness was considerably larger in the T–L orientation than in the L–T orientation at low D K region,thus it is believed that the difference in crack closure was induced from fracture surface roughness.It should be noted that the enhanced FCP rates in 3%NaCl aqueous solution observed in the d a /d N –D K relationships are still seen.This indicates that there ex-ists an environmental effect on FCP behaviour.As anFig.3.Microstructures:(a)base metal,(b)heat affected zone (HAZ),(c)weld metal.94M.Akita et al./Materials and Design 27(2006)92–99example,Fig.6reveals SEM micrographs of fracture surfaces in air and 3%NaCl aqueous solution.At low D K region,the appearance of fracture surfaces is similar in both environments,but at high D K region some inter-granular fracture can be seen on the fracture surface in 3%NaCl aqueous solution.3.3.FCP behaviour of welded jointsThe FCP behaviour for the N,H and W specimens is shown in Figs.7–9,respectively.For comparison,the FCP behaviour for the base metal is also included in those figures.N-specimen (Fig.7)In air,the FCP rates are nearly the same as those for the base metal in low D K region.With increasing crack growth,fluctuation of FCP rate becomes pronounced and FCP rates decrease temporar-ily around D K =20MPa m 1/2,then increase and tend to approach the FCP behaviour for the base metal.Such a complicated FCP behaviour appears to be due to resid-ual stress and hardness changes induced by welding.In 3%NaCl aqueous solution,the FCP rates are con-siderably lower than those for the base metal and fluctu-ation of FCP rate is not seen,being due to a higher initial D K level employed in the experiment.Fig.6.SEM micrographs showing fracture surfaces of base metal.M.Akita et al./Materials and Design 27(2006)92–9995H-specimen(Fig.8)In air,the FCP rates are lower than those for the base metal,particularly in low D K re-gion and with increasing D K gradually approach and then coincide with the FCP rates for the base metal at high D K region.This indicates a higher FCP resistance of HAZ than the base metal.In3%NaCl aqueous solution,the FCP rates are fas-ter than those in air,which becomes more pronounced with increasing D K.When compared with the data for the base metal,the FCP rates are lower in the entire D K region,particularly remarkable at low D K region.W-specimen(Fig.9)The overall FCP behaviour is similar to that of H-specimens.In air,the FCP rates are considerably lower than those for the base metal in low D K region,then gradually approach and eventually coincide with the FCP rates for the base metal at high D K region.This also indicates a higher FCP resistance of the weld metal than the base metal.In3%NaCl aqueous solution,the FCP rates are nearly consistent with those in air at low D K region, but become faster with increasing D K.When compared with the data for the base metal,the FCP rates are sig-nificantly lower in low D K region and then rapidly in-crease and coincide with the FCP rates at high D K region.3.4.Fracture surface analysisSEM micrographs of fracture surfaces in air are shown in Fig.10.As can be seen in thefigure,the oper-ative micromechanisms are the same in all the welded specimens:ductile transgranular at low D K region,while striation at high D K region.On the other hand,fracture micromechanisms oper-ated in3%NaCl aqueous solution were different from those in air and will be discussed later.4.Discussion4.1.Factors influencing FCP behaviour of welded joints in airAs seen in Fig.7,in air the N-specimens showedfluc-tuations of FCP rate and a remarkable decrease in FCP rate around D K=20MPa m1/2.It has been well known that residual stress plays a significant role in the FCP behaviour of welded joints.Fig.11represents the FCP behaviour and residual stress distribution in the N-spec-imens as a function of crack length in both environ-ments.It should be noted that the remarkable decrease of FCP rate in air occurs as the crack reaches HAZ. On the other hand,compressive residual stresses de-crease monotonously with increasing crack length,indi-cating that the residual stress distribution does not correspond to the remarkable decrease in FCP rate just before reaching HAZ.The similar results have been re-ported in laser welded butt joints,which was due to a sudden hardness change at the boundary between the base metal and HAZ[7].At low D K region,in spite of the presence of compressive residual stresses,the FCP rates for the welded specimens are nearly the same as those for the base metal(see Fig.7),this is because the compressive residual stress of approximatelyÀ270 MPa was detected in the base metal,which is equivalent96M.Akita et al./Materials and Design27(2006)92–99to the compressive residual stresses in the welded specimens.The results in3%NaCl aqueous solution did not showcomplicated FCP behaviour as seen in air.This is due to a higher initial D K level employed in the experiment, thus the effect of HAZ becomes relatively small.In the H and W specimens,the cracks propagated within HAZ and the weld metal,pres-sive residual stress of approximatelyÀ300MPa and ten-sile residual stress of100MPa were present at the top and bottom surfaces in HAZ,respectively.Residual stress in the weld metal could not be measured,but it is assumed that similar residual stresses would beFig.10.SEM micrographs showing fracture surfaces of all welded specimens in air.M.Akita et al./Materials and Design27(2006)92–9997present.Although it is not easy to understand the effect of residual stress in such a case,pressive on one side and tensile on the opposite side,it seems that the FCP behaviour is affected by compressive residual stress rather than tensile residual stress,because the absolute value of the former is significantly larger than that of the latter.In addition to residual stress,deflection of crack path was much more pronounced in HAZ and the weld metal due to grain growth and solidification microstructure,respectively,which also contributes the higher FCP resistance of HAZ and the weld metal than the base metal.4.2.FCP behaviour after allowing for crack closure The FCP behaviour characterized in terms of D K effis shown in Fig.12.As can be seen in the figure,in air the d a /d N –D K effrelationships for all the welded specimens are almost identical to that of the base metal.Therefore,the differences among the welded specimens and be-tween the welded specimens and the base metal observed in the d a /d N –D K relationships are attributed to crack closure that was induced by residual stress and fracture surface roughness.In 3%NaCl aqueous solution,the d a /d N –D K effrela-tionships in all the welded specimens are nearly the same,thus the difference among the welded specimens observed in the d a /d N –D K relationships is also attrib-uted to crack closure.However,when compared with the d a /d N –D K effrelationships in air,the FCP rates in 3%NaCl aqueous solution are significantly faster in the region of D K eff>15–20MPa m 1/2,which indicates that an environmental effect exists in this region.4.3.Effect of corrosive environment on FCP behaviour of welded jointsAs seen in Fig.12,the FCP rates of all the welded specimens were still faster at high D K effregion in 3%NaCl aqueous solution,suggesting that operative micromechanisms would be different from in air.Therefore,fracture surfaces were examined in detail using SEM.SEM micrographs of fracture surfaces at high D K region where the enhanced FCP rates were observed are shown in Fig.13.At low D K region where the same FCP rates were observed in both environments,the fracture surfaces in 3%NaCl aque-ous solution revealed ductile transgranular,which was the same as in air.At high D K region,however,a significant fraction of intergranular fracture or quasi-cleavage fracture can be seen (Fig.13),indicat-ing clearly that different fracture micromechanisms from in air have operated in 3%NaCl aqueous solution.SCC,hydrogen embrittlement and intergranular cor-rosion are believed to be the possible causes of brittle nature in 3%NaCl aqueous solution.However,the effect of SCC would be negligible,because it has been indi-cated that ferritic stainless steels had very low suscepti-bility to SCC even in a highly concentrated chloride solution [8].In order to confirm the resistance to SCC of the present 444stainless steel,SCC tests have been performed using CT-specimens in 3%NaCl aqueous solution,but SCC did not take place.On the other hand,it has been pointed out that hydrogen embrittlement oc-curred under cathodic potentials in high purity ferritic stainless steel [9].However,hydrogen embrittlement would not take place in the present case,because of a low hydrogen-produced environment,i.e.3%NaCl aqueous solution.Therefore,it is believed that inter-granular corrosion would be the cause for the enhanced FCP observed in 3%NaCl aqueous solution,because it has been indicated that HAZ became sensitive to inter-granular corrosion in ferritic stainless steel weldments [8],and thus welded joints would have increasing pro-pensity of intergranular fracture compared with the base metal.The weld metal is an austenitic stainless steel,type 309L,which also showed enhanced FCP at high D K effregion.This would be attributed to hydrogen embrittle-ment of martensitic phase transformed from austenitic phase [10].Fig.13.SEM micrographs showing fracture surfaces of all welded specimens in the FCP regime enhanced by 3%NaCl aqueous solution.98M.Akita et al./Materials and Design 27(2006)92–995.ConclusionsFatigue crack propagation(FCP)behaviour for large cracks was studied on welded joints of a ferritic stainless steel,type444,in air and in3%NaCl aqueous solution.The obtained results were discussed on the basis of residual stress,crack closure behaviour and fracture surface analysis.The conclusions can be made as follows:(1)In air,the welded specimens of which FCP direc-tion was perpendicular to the weld line showed a complicated FCP behaviour with frequentfluctua-tions of FCP rate.When the crack reached HAZ, the FCP rates were significantly decreased and then monotonously increased.When the cracks propa-gated within HAZ and the weld metal parallel to the weld line,the FCP rates for both specimens were almost identical and lower than those for the base metal in the entire D K region.(2)The FCP rates of all the welded specimens in3%NaCl aqueous solution were enhanced com-pared with those in air,being more pronounced with increasing D K.(3)In air,after allowing for crack closure,all thewelded specimens showed nearly the same FCP behaviour,indicating that the observed differences in the FCP rate characterized in terms of D K were attributed to crack closure.(4)The FCP rates in3%NaCl aqueous solution werestill faster at high D K effregion than those in air after allowing for crack closure,where extensive inter-granular fracture or quasi-cleavage fracture was seen.AcknowledgementsThe authors thank Mr.Y.Asano,Mr.T.Hayakawa, Mr.M.Itoh and Ms.C.Ohmori for their experimental assistance.References[1]Nakazawa T,Suzuki S,Sunami T,Sogo Y.Application of high-purity ferritic stainless steel plates to welded structures.ASTM STP1980;706:99–122.[2]Redmond JD.Toughness of18Cr–2Mo stainless steel.ASTMSTP1980;706:123–44.[3]Kimura Y,Yagasaki Y,Kunio T.Initiation process of corrosionfatigue cracks in stainless steels.Trans Jpn Soc Mech Eng 1984;A50-1:33–40.[In Japanese].[4]Nakajima M,Akita M,Tokaji K,Shimizu T.Fatigue crackinitiation and early growth behavior of a ferritic stainless steel in laboratory air and in3%NaCl aqueous solution.JSME Int J,Ser A2003;46–4:575–81.[5]Krysiak KF.Weldability of the new generation of ferritic stainlesssteels-update.ASTM STP1980;706:221–40.[6]Kikukawa M,Jono M,Tanaka K,Takatani M.Measurement offatigue crack propagation and crack closure at low stress intensity level by unloading elastic compliance method.J Soc Mater Sci Jpn 1976;25:899–903.[In Japanese].[7]Minagi A,Tokaji K.Fatigue crack propagation of laser weldedbutt joints.Fracture mechanics:applications and challenges.In: Proceedings of the13th european conference on fracture (ECF13).San Sebastian,Spain;2000[CD-ROM].[8]Kowaka M.The corrosion behavior of ferritic stainless steels.JSoc Mater Sci Jpn1974;23:924–35.[In Japanese].[9]Koterazawa K,Uchida H,Ohshiba H.Effect of tensile orientationon hydrogen embrittlement in single crystals of high purity ferritic stainless steel.J Soc Mater Sci Jpn1993;42:983–9.[In Japanese].[10]Endo K,Komai K,Murayama S.Influence of ClÀconcentrationon corrosion fatigue crack growth of an austenitic stainless steel.Trans Jpn Soc Mech Eng1982;48:1489–95.[In Japanese].M.Akita et al./Materials and Design27(2006)92–9999。
材料英语词汇F-J
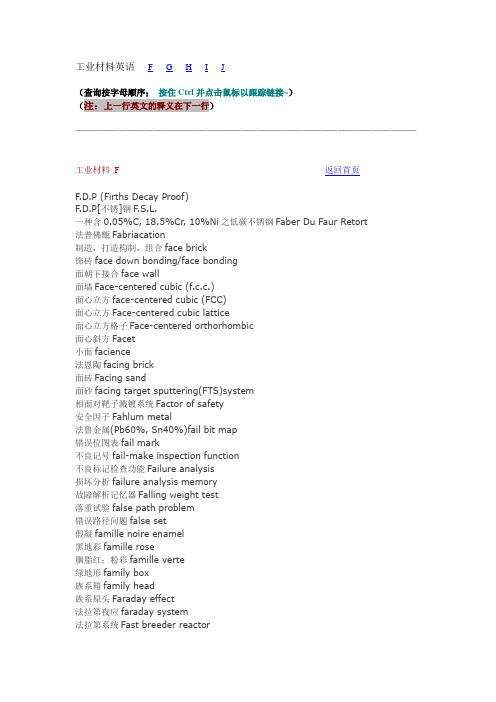
工业材料英语F G H I J(查询按字母顺序;按住Ctrl并点击鼠标以跟踪链接~)(注:上一行英文的释义在下一行)———————————————————————————————————————工业材料F返回首页F.D.P (Firths Decay Proof)F.D.P[不锈]钢F.S.L.一种含0.05%C, 18.5%Cr, 10%Ni之低碳不锈钢Faber Du Faur Retort法普佛甑Fabriacation制造,打造构制,组合face brick饰砖face down bonding/face bonding面朝下接合face wall面墙Face-centered cubic (f.c.c.)面心立方face-centered cubic (FCC)面心立方Face-centered cubic lattice面心立方格子Face-centered orthorhombic面心斜方Facet小面facience法恩陶facing brick面砖Facing sand面砂facing target sputtering(FTS)system相面对靶子溅镀系统Factor of safety安全因子Fahlum metal法鲁金属(Pb60%, Sn40%)fail bit map错误位图表fail mark不良记号fail-make inspection function不良标记检查功能Failure analysis损坏分析failure analysis memory故障解析记忆器Falling weight test落重试验false path problem错误路径问题false set假凝famille noire enamel黑地彩famille rose胭脂红;粉彩famille verte绿地形family box族系箱family head族系原头Faraday effect法拉第夜应faraday system法拉第系统Fast breeder reactor快滋生反应,快增殖反应器Fast neutron快中子(能量超过0.1Mev)fast traverse快速通过速度fat clay富黏土(从土木)Fat sand高黏土砂Fatigue corrosion疲劳腐蚀Fatigue crack疲劳裂痕Fatigue fracture疲劳破裂Fatigue limit疲劳限Fatigue notch factor疲劳凹痕因子Fatigue test疲劳试验Fatigue test machine疲劳试验机Fatigue tester疲劳试验机fault缺陷fault analysis故障分析fault circuit故障电路fault coverage故障概括率fault dictionary故障辞典fault simulator故障仿真器Fayalite铁橄榄石,铁硅酸盐Faying surface1 接合面2 待接合面Feal费尔合金(25%Al,其余为Fe有良好制振性)feather combing梳花feather marking羽状痕迹feed gums种籽胶Feed ring环冒口Feed riser冒口Feed water给水feeder饲机feeder channel饲槽feeder gate饲料闸Feeding rod冒口搅棒Feldspar长石feldspathic glaze长石岫feldspathoid似长石felsophyne硅长斑石felsparfeldsparFeltmetal金属纤维毡Feran包铝钢条Fermi level费米准位(原子)Fermi surface费米面(原子)Fermium (Fm, 100)镄Fernichrome费理铬(一种能封入铅玻璃内之材料,含37%Fe,30%Ni,25%Co,8%Cr)Ferrari cement 佛拿里水泥Ferric oxide三氧化二铁ferrimagnetism亚铁磁性Ferrious material铁系金属Ferrite1 肥粒铁2 磁性氧化物ferrite (ceramic)1肥粒铁2磁性氧化物Ferrite grain size肥粒铁晶粒度Ferrite martensite肥粒形麻田散体Ferritic cast iron肥粒铁铸铁Ferritic decarburized depth脱炭层之肥粒铁深度Ferritic stainless steel肥粒铁不锈钢ferro boron硼铁Ferro-alloy (ferroalloy)合金铁ferro-bronge铁青铜(8%Fe, 0.7%Cr,其余为Cu)Ferro-chrome铬铁Ferro-electric materials铁电材料(如BaTiO3)Ferro-manganese锰铁Ferro-molybdenum钼铁Ferro-phosphorus磷铁Ferro-silicon硅铁Ferro-titanium钛铁Ferro-tungsten钛铁Ferro-vanadium钨铁Ferrochrome-silicon硅铬铁ferroele ctric强电介体(从电机)ferroelectric thin film铁电薄膜强诱电体薄膜Ferrograph磁滞仪Ferrolum包铅钢ferromagnetic强磁的Ferromagnetic domain wall铁扇区壁Ferromagnetic spinel铁磁性尖晶石ferromagnetism铁磁性,强磁性Ferromanganese-silicon硅锰铁(Ferron福朗(含35%Ni, 15%Cr,其余为铁的一种抗氧化合金)Ferronickel镍铁Ferrose-forric oxide磁性氧化铁(Fe3O4)Ferroselenium硅铁Ferrostan电镀马口铁(镀锡冷卷钢)ferrous alloy铁合金Ferrous metallurgy钢铁冶金学Ferrous oxide氧化亚铁Ferrox cube软磁磁体族Ferry福里合金(一种含55%Cu, 45%Ni之高电阻材料,类似康史登钢)Fertile material 可育材料Fery radiation pyrometer费氏辐射高温计fetrafluoroethylene oxide氧化四氟乙烯Fettling修整(1 铸件加工前之修整2 炉衬之修整)Fiber metal纤维金属Fiber reinforced plastice (FRP)纤维加强塑料Fiber stress纤维应力Fiberfax岩绒,金刚纤维fiberglass玻璃纤维fibre, ceramic陶瓷纤维Fibrous falc纤维状滑石Fibrous structure纤维组织Fick's first law菲克(扩散)第一定理Fick's law菲克[扩散]定律Fick's second law菲克(扩散)第二定理fiducial mark基准标记Field batch weight工地盘重Field control工地控制Field effect transistor (FET)场效应晶体管field-drain pipe工地排水管figured glass压花玻璃Filberflow纤维流纹filed programmable gate array现场可程序闸门数组filed programmable interconnect 现场可程序互接filler填充料Filler metal熔填金属fillet接合剂渗出痕迹,接合轮廓film strength干釉强度Film test薄膜试验filter(活性炭)过滤器filter block泸砖filter candle泸筒filter cke泸饼filter cloth滤布Filter press压滤机filtration equipment with coagulation凝聚过滤设备fin缝翅final energy magnet终极能量磁铁final polishing最后加工抛光仕上研磨final test最后测试final wafer test晶圆后端测试Fine aggregate细骨材fine alignment精细对准Fine gilt薄层镀金Fine grain细晶粒,细[颗]粒fine grinder细研机fine grinding细磨Fine pearlite微粒波来铁fine pitch精细节距fine powder超微粒Fine-grained steel细晶粒钢fineness moldulus细度模数Fineness of cement水泥细度Finess number粒度(铸砂)Finger gate指形铸口finial屋脊饰fining澄清Finishability饰面难易度Finished steel精炼钢Finishing impression完成型Finishing machine整面机Finishing mill完成巴机Finishing roll未巴辊Finishing temperature完成温度Fink process芬克浸铝法Finkle-Mchr process芬克穆尔法finl set终凝finyl chloride polymers氯乙烯聚体fir brick火砖fir resistance耐火性fire back垫[背]火砖fire box火箱Fire brick耐火砖fire bridge火坝Fire clay耐火泥fire crack热裂fire finished火修光Fire magnesite-chrome brick 烧镁铬砖fire mark火痕fire pillar火柱fire polished火焰磨光fire proofing tile坐火瓦Fire refining火法精炼Fire sand耐火砂Fire welding固态接合,接火Fire-box steel火箱钢Fire-cracking热裂firecaly火黏土Fired chrome brick烧铬砖Fired magnesite brick烧镁砖Firestone耐火石firing1烧成2点火firing expansion烧制膨胀firing range烧制范围firing shrinkage烧[成][收]缩firingtemperature烧成温度first bonding第一接合,第一压接First coat1 底漆2 第一涂层first dislocation generation 初次发生位错First stage annealing第一段退火firsts一级品Firth hardometer费氏硬度计Firty耐火性Fish scale鳞状破面fish-scale鳞剥fisheyes鱼眼(因氢脆性之裂开面)Fishtail鱼尾式Fissile material可分裂材料Fission分裂Fission fragment分裂碎片Fission poison分裂毒物Fission product分裂产物Fissionable material可分裂材料Fissium分裂碎片合金fitting配件five points thickness variation5点厚度变动Fixed carbon固定碳fixing block受钉砖块fixture board夹具基板Fizeau interferometer method菲氏干涉计法flabby cast软铸物Flake1 小片2 裂纹3 白疵Flake graphite片状石墨Flake graphite cast iron片状石墨铸铁Flaking剥落Flame annealing火焰退火Flame cleaning火焰清除法Flame cutting火焰切割Flame hardening火焰硬化flame photometer焰度计flame plating焰镀[法]Flame scaling焰烤锌皮法flame sensor火焰传感器Flame softening火焰软化Flame spectro fluorimetry火焰光谱分析Flame spraying火焰喷涂Flame straightening火焰矫直Flame strengthening火焰强化Flame-plating火焰涂层flame-spraying焰喷[法]Flame-tempering火焰回火flameless combustion无焰燃烧Flanging折缘Flappin [of copper]翻搅flare bed发焰道Flare test顶进定量变形试验(与drift test同)Flaring扩口Flash飞边,溢边Flash and fire point test闪点及燃点试验flash lamp annealer闪光灯退火处理机flash memory E/W cycle test system 闪存删除写入周期测试系统Flash point闪点Flash radiography闪烁放射性摄影Flash roasting闪燃煅烧flash shield溢边护罩flash wall闪火墙Flash welding闪电熔接Flash-butt welding闪电对头熔接Flask1 砂箱2 烧瓶flat bar1 扁条2 扁条钢flat collet平面吸具Flat dies平模Flat grain平纹flat zone length热平坦区长度Flat-die forging平模锻造,平模锻件Flat-die hammer平模锤flat-ware浅皿flatness平面度flatness quality area平面度适用区域Flats扁钢(1/8~1/4吋厚两呎以卷宽之卷钢片)Flattener矫平机具flatting平整[法]Flemish bond法式砌法(从土木)Flexibility凸挠性flexural strength抗弯强度Flexure tests弯曲试验Flint火石,燧石flint clay燧石黏土Flint glass火石玻璃flint shot燧石粒flip chip bonder倒装片接合机flip chip bonding侧装片接合float glass process浮制玻板法float polishing浮动抛光Float test index浮游试验指数floatation equipment漂浮分离设备floater浮舟(玻)floating agent浮剂Floating die浮动模floating license浮动许可证Floating zone crystal growing浮融带晶体生长法floating zone melting method 浮动区熔法Floating-zone refining浮融带纯化floc testwater testflocculant絮凝剂Flocculation絮凝,凝聚Flong纸型(活字版铸版用)flood casting泄注法floor brick地砖Floor molding地模法floor planner平面规划器floor quarryquarry tilefloor tile铺地砖Flotation浮选Flow ability流动性Flow ability of sand铸砂流动性flow blue霁青Flow brightening亮锡法Flow coating流动施膜Flow line1 加工流程2 锻造流线Flow line network流线网,流程网flow machine致流机Flow off溢流口flow pattern defect流体图案缺陷Flow spinning切刀旋转法Flow stress流度应力flow table流度台flow test流度试验Flow through溢流道Flow welding熔流熔接Fluctuating stress波动应力flue gas烟道气fluid-energy mill流体能磨Fluidity流体性Fluidity index流体性指数fluidized bed流体化床Fluidized sand流动性铸砂Fluidized sand molding流动性铸砂造模法fluidized-bed coating流体床法护膜Fluon氟罗央(PTFE的商业名称)Fluorescence荧光Fluorescence analysis荧光分析Fluorescence particle inspection烛光颗粒检验Fluorescent material荧光材料Fluorescent penetrant test荧光透入剂试验fluorite萤石Fluorite (fluorspar)萤石Fluoroscopy荧光分析术fluorspar萤石Flush quenching闪激卒火flux1助熔剂2焊剂3熔剂4通量(磁电)flux factor 熔剂因子flux line玻膏面Flux-covering熔剂覆盖Fluxing熔剂处理Fly ash飞灰flying arch跳顶拱Flying saw随动锯机Flying shear随动剪机Flying spot microscope飞点式显微镜foam concrete泡沫混凝土foam glass泡沫玻璃foamed clay起泡黏土foamed concrete起泡混凝土Foaming起泡focal plane焦点平面focal plane deviation焦点平面偏差Fog quenching喷雾卒火Foil箔Foil heat treatment箔袋热处理Fold折痕Folding defect折迭瑕疵Follow board嵌模板Fool's gold愚人金(黄铁矿FeS2)Foolner process福药法(磷酸盐处理)foot print脚印Footing基脚Foppl test福贝耳[硬度]试验Forbidden band禁带(原子)force fill process加压填埋空隙forced draft强制通风Forced gas convection强制气对流Fore blow前期吹风Fore hand welding前进熔接Fore hearth前炉Foreign atom外来原子Forge hammer锻锤Forge iron锻铁Forge rolls锻辊Forge welding锻造熔接,锻接Forgeability锻造性Forged steel锻钢forging1锻造2锻件Forging dies锻模Forging machine锻机Forging, closed-die1 闭模锻造2 闭模Forging, cold冷模Forging, flat-die1 平模锻造2 平模Forging, hammer锤锻Forging, hand手工锻造Forging, high-energy-rate高能率锻造Forging, high-velocity高速锻造Forging, hot热锻Forging, machine机械锻造Forging, open-die1 开模锻造2 开模锻件Forging, pancake 扁圆锻件Forging, press压机锻造Forging, roll轧机锻造Forging, smith手工锻造Forging, upset1 锻粗锻造2 锻粗锻件fork铁叉form chamfering整形去角取面Formability成形性formal verification正式验证formatter格式编制器Forming成形forming gas组成气体Forming, bulge胀大成形Forming, compression压缩成形Forming, contour roll型辊成形Forming, creep潜伸成形Forming, drop hammer落锤成形Forming, electrohydraulic 电液成形Forming, electromagnetic 电磁成形Forming, explosive爆炸成形Forming, high-energy-rate 高能井成形Forming, hydraulic液压成形Forming, magnetic pulse电磁震动成形Forming, multiple-slide 多滑块成形Forming, press压机成形Forming, press-brake折条机成形Forming, radial-fraw径拉成形Forming, rubber-diaphram 橡胶膜成形Forming, rubber-pad橡胶垫成形Forming, rubber-punch橡胶冲成形Forming, shrink收缩成形Forming, stretch拉伸成形Forming, three-roll三辊成形Forming, wacuum真空成形Forming, wire线料成形Forms模板Forrester flotation machine富尔斯特浮还机forsterite镁橄榄石Forsterite (Mg2SiO4)硅酸镁石forward annotation正向批注Forward extrusion顺向挤制Forward spinning顺向旋挤Forward welding前进熔接Foucault current method傅科电流法Fouling积垢(船底海藻贝壳)fouling index(FI)污浊指标(FI)Fouling resistance防垢性(铅底)Foundation基础Founder铸工Founding铸造Foundry1 铸造2铸造场Foundry coke铸焦Foundry defects铸疵Foundry ladle铸造浇斗,铸造盛通Foundry losses铸造损耗Foundry pig铸造生铁Foundry pig iron铸造用生铁Foundry returns回炉料Foundry sand铸砂Foundry type metal手植活字合金four(point)probe method四(点)探针法Four-high mill四辊巴机,四重式轧机four-way type四方向方式Fourier transform infrared spectroscopy 傅立叶转换红外光谱学four(point)probe method四(点)探针法Fractional crystallization分段结晶Fractography断口型像学Fracture mechanics破断力学,破坏力学Fracture stress破断应力Fracture surface破断面Fracture test破断试验fracture toughness破裂韧性Fracture transition脆断转移[温度]Frame filter press框式压泸机frame memory视帧记忆器;图框记忆器Frame work structure构架结构framework架构Francium (Fr, 87)金法Frand-Read source法一瑞式[差排]源Frank partial dislocation法兰克部份差排Frary metal法拉钡合金(一种铅钨钡合金)Free carbon游离碳Free cementite游离雪明碳铁free cutting brass易切黄铜free cutting bronze易切青铜Free cutting steel易切钢Free electron自由电子free energy自由能Free ferrite游离肥料铁Free lime游离石灰Free water游离水份Free-cutting metal易切金属free-flow bender开放弯曲机freeze-casting冻铸法freezing冷凝,冻结凝固freezing and thawing test冻结融解试验Freezing cuve冷凝曲线Freezing method冷凝法Freezing point1 凝固点;2 冰点Freezing range凝固范围French chalk法兰西白呈(即滑石3MgO4SiO2、H2O)French metal 法兰西金属(一种精炼锑)French process法兰西法(一种提锌法)Frenkel defect佛兰克缺陷(晶格缺陷)Fresh water淡水Fretling corrosion[紧压]磨蚀,移擦腐蚀friction element摩擦组件Friction oxidation摩擦氧化friction pressfriction-screw pressFriction sawing摩擦锯切Friction screw press摩擦螺杆压机Friction welding摩擦熔接Frictional loss摩擦损耗frifluoroacetylnitrile亚硝酸三氟乙酰Fringe晕纹frit熔块;玻料fritted glass ware玻釉玻璃器fritted glaze熟釉;玻料釉fritted porcelain玻料瓷Fritting1 烧结(同sintering)2 上釉料frizzling绉缩(陶)frog ring辙叉环Fromont test傅瑞孟[冲击]试验front end design前端设计front side reference method前侧基准法Front slagging前渣法Frontier alloy佛兰提合金(一种铝锌合金)frost glass磨砂玻璃Froth flotation泡漆浮选Fry's reagent傅蕊族剂fuel cell燃料电池fuel cell stack燃料电池堆Fuel cycle燃料循环Fuel element燃料组件Fuel fabrication燃料构制Fuel oil燃料油Fuel ratio燃料比Fuel reprocessing燃料再处理Fugacity (fugasity)1 挥发度2 挥发性Full annealing完主退火full automatic growing furnace 全自动成长炉full cutting全切割Full hardening完全硬化Full insert整体嵌件Full mold process全模法full site全体区分地段Full-killed steel全静钢Full-mould casting process金模铸造法Fuller半圆型锤fuller earth漂白土Fuller's ideal curve富乐理想曲线Fullering敛缝,填隙fullering bar填隙棒Fullering fmpression敛缝型Fully-killed steel全静钢Fulminates雷酸盐(不稳定易爆材料)fume烟气function test功能测试Functional ceramics功能陶瓷functional description language功能记述语言functional schematic editor功能检图编辑器Fundamental vector Furnace 基本向量炉Fundation translation vector基本平移向量funerary ware明器furnace angle炉体倾斜角furnace annealer电热炉退火处理机furnace lift travel炉移动行程Furnace lining炉衬furnace tube cleaning equipment炉心管洗涤设备Furnace, baking烘焙炉furnace, batch [type]批式炉furnace, bell-type钟罩式炉furnace, bogie hearth钟罩式炉,拖车式炉furnace, box箱式炉Furnace, car bottom车底式炉Furnace, chain type链式炉Furnace, continuous连续式炉Furnace, continuous-strand连绩回式炉Furnace, conveyor (conveyer)输送带炉Furnace, direct arc直接电弧炉Furnace, high frequency高周波电炉Furnace, holding保温炉Furnace, indirect arc间接电狐炉Furnace, lift off cover离盖式炉Furnace, overhead conveyer高吊输送炉Furnace, pit坑式炉Furnace, preheating预热炉Furnace, pusher推式炉Furnace, remelting再泰炉Furnace, roller hearth滚子炉Furnace, rotary-hearth转膛炉Furnace, screw feed螺旋送料炉Furnace, slot-type槽式炉Furnace, tunnel隧道炉furnace, walking beam动梁炉fuse data熔丝资料fuse link链丝熔丝Fuse-quench process熔化急冷法Fused alumina熔凝铝氧Fused quartz熔凝石英Fused sand熔砂fused silica熔硅石Fused zone泰融带Fused-cast refractory熔铸耐火材料fused-salt electrolysis泰盐电解Fused-silica frick熔凝硅砖Fused-silica refractory泰凝硅质耐火材料Fusible alloy易熔合金Fusible pluger易熔塞Fusing pyrometer融点高温计fusion1 熔化,熔解2 熔合(核子)fusion casting熔铸法Fusion of packing materials填料熔合Fusion penetration熔融穿入Fusion point熔融点Fusion range熔融区Fusion sawing熔融锯Fusion temperature熔融温度Fusion welding熔融泰接fusion-flow test熔流试验(搪瓷)fuzzy texture疏松结构(搪瓷)工业材料G返回首页g-line stepperg线步进机G-valueG值gable tile山墙瓦gable wall投料墙gadget支架gaffer吹制领班gage (gauge)1量规2号规(线) 3样板gage length标距gagger撑条gaging规测gain refiner微晶剂galena方铅矿(Pb S)gall浮硝galleting碎瓦片(陶)galling1(压紧面间)擦伤2吸住,紧贴gallium金家gallium antimonide锑化金家gallium arsenide砷化金家(Ga As)(一种半导体)gallium phosphide磷化金家(Ga P)(一种半导体)Gallo tannin acid没食子酸galvanic action[丹尼尔]电池作用galvanic cell化学电池galvanic corrosion电池腐蚀Galvanic deposit电[池性]沈积galvanic potential电转电位galvanic series电转序(电转电动势次序)galvanized iron镀锌铁galvanized steel sheet镀锌钢片galvanized steel wire镀锌钢丝galvanizing热镀锌法galvanizing热浸镀锌galvanizing电积镀锌法galvannealing镀针退火galvanostatic polarization curve电流恒定极化曲线Galvznizing镀锌法galvznizing embritllement镀锌脆性gamma brassγ黄铜,咖玛黄铜gamma dispersionγ色散,咖玛色散gamma ironγ铁gamma loopγ区,咖玛区gamma radiationγ辐射,咖玛辐射gamma rayγ射线gamma solid solution咖玛固溶体gamma structure咖玛结构gamma-radiographyγ射线照相术,咖玛射线照相术gamma-ray fluoroscopy γ玛对线荧光照相检验gamma-rays咖玛射线(波长小于0.5A之电磁辐射线)gang bonding多端子接合gangue脉石ganister硅石gap frame press弓架压机gap grading不连续粒度Gardner mobilomete贾得纳流度计Gareis—Endell plastometer贾恩塑性计garnet石榴石gas adsorption method气[体]吸附法gas box瓦斯筒收纳箱gas carburizing气体渗碳法gas centrifuge process气体离心法gas classification气体分级法gas coke煤气焦碳gas concrete充气混凝土gas constant气体常数gas cutting焰割gas cyaniding气体氰化法(碳氮处理)gas feed system瓦斯输送系统gas hole气孔gas law体定律gas permeability透气性gas pickling气(体)浸(渍)法gas porosity气体孔隙率gas pressure bonding高温气的等压法gas source molecular beam epitaxial growth system 瓦斯源分子束磊晶生长系统gas turbine燃气涡轮机gas welding气体熔接,气焰熔接gas-cooled reactor气体冷却反应器gas-cooled reactor进步型气冷反应器gas-shielded arc welding气护电弧熔接gaseous diffusion process气体扩散法gasket corrosion (crevice corrosion)隙腐蚀(裂隙腐蚀)gassing of copper铜之汽损gassy surface疏松结构gate1进模口2闸gate array闸门数组gate cutting切断成型铸模树鲁流导闸门之残留树脂gate insert piece插入闸门之分割用隔片gate level description闸门位阶记述gate stick浇道棒gate value栅型阀gather蘸料gather hole蘸料口gating system浇注系统Gaudin's equation高丁公式gauge曲脚gauge factor磨度计因子gauge length标距gauging of cement水泥稠度标准化Gauss高斯(磁感应或磁场强度单位)Gazal process盖撒法Ge-Si alloys锗硅合金(热电材料)gear bronze齿轮青铜Geiger counter盖氏计数器Geiger-Mueller counter盖格-密勒计数器gel凝胶,乳胶体gelatine, gelating明胶general attack主面侵蚀geochemistry地质化学Geomagnetic field地磁场Georgian glass方网玻璃Gerber's law盖柏定律(有关疲劳行为)German silver (nickel silver)德银(α相之铜镍针合金);白铜,洋银germanium nitride 氮化锗germanium oxide氧化锗Germanuium (Ge, 32)锗Germination伟晶作用Gero degassing process吉罗去气法Getter收气剂gettering吸器,除器getting采掘Ghost band (ghost line)魔带,魔线Gibbs free energy吉布自由能Gilard &Dubrul factor吉杜因子(玻)Gilding镀金gilding bronze拟金黄铜(含5%Zn之黄铜)Gillmore needle吉摩针(泥)Gilt包金件(以薄金属被覆之银或其它非铁金属)glaceramic玻陶Glaging上釉glass玻璃glass enamel玻璃珐琅彩料glass eye玻泡(玻)Glass fiber玻璃纤维glass fibre玻璃纤维glass fost玻白glass frit sealing equipment玻璃料密封装置glass house玻璃工场Glass naol玻璃绒glass to metal seal玻金封点(玻)glass transition temperature玻璃转换温度glass-bonded mica玻结云母glass-coated steel & glass-lined steel玻衬器皿glass-forming engobe玻化化妆土Glassy (amorphous)玻璃质(非晶质)glaze釉(陶)glaze fit釉适性(陶)glazer火焰磨光炉;磨光机glazes釉彩glazing上岫;施釉(从化工)Glide plane滑动面Glo-crack荧光示裂法global总体global alignment全晶圆对准global backside indeal range总体背面理想范围global backside variation总体背面理想范围global flatness总体平面度global leveling全晶圆调平global tilting全晶圆倾斜转动Globar格罗棒(碳化硅电热棒)Globular cenentite球状雪明碳铁Globular pearlite球状波来体Globurizing球状化glory hole小烘炉gloss光泽gloss point岫熔点glost0fire岫烧Glow discharge nitriding辉光放电氮化glut arch焚口(陶)GO/NO-GO test性能良?判定试验gob玻璃膏球gob process玻器制法Goggles护目镜Gold (Au, 79)金gold bronze金色青铜(α\铝青铜,今3-5%Al其余为Cu)gold decoration 金饰(陶)Gold leaf金叶(3-5 X10-6inch原金箔)gold rubyglass金赤玻璃gold scouring刮亮Goldchmidt Thermit process戈德史密特铝热法goldstone glaze金路岫Gonell air elutriator哥涅淘析机Goniometer1 测塞器2 测向仪Goodmans law古德曼定律(疲劳行性)Goskar dryer哥斯卡干燥机Gothic pitch哥德斜度Gottignies kiln哥近尼[多隧]窑Gouge半圆凿gouging test抗磨试验(搪)Gradation级配grade等级Graded aggregate级配骨材Graded standard sand级配标准沙Gradient梯度,倾差gradient freeze method凝固温度梯度法Gradient mold斜度模铸锭模Grading分级grading analysis级配分析Grading curve级配曲线grading incidence interferometer method 倾斜入射干涉计法Graduating刻度grain晶粒Grain boundary晶[粒境]界Grain boundary attack晶界侵蚀Grain boundary reaction晶界反应Grain coarsening晶粒粗化Grain coarsening temperature晶粒化温度Grain contrast晶粒[反光]对比Grain fineness number颗粒细度grain grit粗粒;磨粒粒度Grain growth晶粒生长grain of maximum size最大魔粒grain percentage魔粒率;磨料百分比Grain refinement晶粒微化Grain refining晶粒微化Grain refining temperature晶粒微化温度Grain size晶粒度,晶粒大小grain size analysis粒度分析Grain structure晶粒组织graininggraining oxideGram-atom克原子Granite花岗石granite ware斑点单轴搪瓷Granodising锌面磷酸盐(黑层)处理granular activated carbon颗粒状活性碳Granular pearlite粒状玻来铁Granular power粒状粉未Granulated slag粒状熔渣Granulation粒状化,造粒Granulation method粒化法,造粒法Graphite石墨Graphite carbon石墨碳Graphite corrosion石墨腐蚀Graphite crucible石墨坩埚Graphite electrode石墨电极Graphite flake precipitation片状石墨析出graphite heater annealer石磨加热器退火处理机Graphite steel石墨钢Graphite, rosette菊花状石墨Graphite-claybrick石墨黏土砖Graphitization石墨化Graphitizer石墨化促进剂Graphitizing anneal石墨化退火Graphitizing inoculant石墨化接种剂Graphtic, embrittlement石墨脆他Grate1 炉条2隔栅Graticude影规(化学镜头中之十字线刻度)Gravimetric method重量法Gravity casting重力铸造Gravity die casting重力金属模铸造法Gravity drop hammer自落锤gravity filter重力过滤器gravity process玻膏滴制法Gravity segregation垂力偏析Gravity sintering重力烧结gray scale pattern recognition灰色标度器案识别Gray [cast] iron灰口铸铁Grazed brick釉砖grazing衬垫表面剥落green生坯Green casting生铸件(未热处理之铸件)Green compact生压胚Green density粉胚体密度(粉未冶金)green house生坯间(陶)Green permeability湿砂透气性Green pressing生压胚Green rot线朽铬线(含高镍之镍铬合金之一种高温腐蚀现象)Green sand 湿模砂Green sand mold湿砂模Green sand molding湿砂造模法green spot深斑green strength生坯强度(陶)Green strentgth湿砂强度Green-tin灰锡(锡在-13.2。
Analytical Methodology For Predicting The Onset Of Widespread Fatigue Damage In Fuselage Structure

ANALYTICAL METHODOLOGY FOR PREDICTING THE ONSET OFWIDESPREAD FATIGUE DAMAGE IN FUSELAGE STRUCTURECharles E. Harris, James C. Newman, Jr.,Robert S. Piascik, and James H. Starnes, Jr.Research and Technology GroupLangley Research CenterNational Aeronautics and Space Administration (NASA)Hampton, VA 23681ABSTRACTNASA has developed a comprehensive analytical methodology for predicting the onset of widespread fatigue damage in fuselage structure. The determination of the number of flights and operational hours of aircraft service life that are related to the onset of widespread fatigue damage includes analyses for crack initiation, fatigue crack growth, and residual strength. Therefore, the computational capability required to analytically predict the onset of widespread fatigue damage must be able to represent a wide range of crack sizes from the material (microscale) level to the global structural-scale level. NASA studies indicate that the fatigue crack behavior in aircraft structure can be represented conveniently by the following three analysis scales: small three-dimensional cracks at the microscale level, through-the-thickness two-dimensional cracks at the local structural level, and long cracks at the global structural level. The computational requirements for each of these three analysis scales are described in this paper.INTRODUCTIONThe ability to analytically predict the onset of widespread fatigue damage in fuselage structures requires methodologies that predict fatigue crack initiation, crack growth, and residual strength. Mechanics-based analysis methodologies are highly desirable because differences in aircraft service histories can be addressed explicitly and rigorously by analyzing different types of aircraft and specific aircraft within a given type. Each aircraft manufacturer has developed mature in-house durability and damage-tolerance design and analysis methodologies that are based on their product development history. To enhance these existing successful methodologies, NASA has adopted the concept of developing an analytical “tool box” that includes a number of advanced structural analysis computer codes which, taken together, represent the comprehensive fracture mechanics capability required to predict the onset of widespread fatigue damage. These structural analysis tools have complementary and specialized capabilities ranging from a nonlinear finite element-based stress-analysis code for two- and three-dimensional built-up structures with cracks to afatigue and fracture analysis code that uses stress-intensity factors and material-property data found in look-up tables or from equations. The development of these advanced structural analysis methodologies has been guided by the physical evidence of the fatigue process assembled from detailed teardown examinations of actual aircraft structure. In addition, NASA is conducting critical experiments necessary to verify the predictive capability of these codes and to provide the basis for any further methodology refinements that may be required. The NASA experiments are essential for analytical methods development and verification, but represent only a first step in the technology-validation and industry-acceptance processes. Each industry user of this advanced methodology must conduct an assessment of the technology, conduct an independent verification, and determine the appropriate integration of the new structural analysis methodologies into their existing in-house practices. NASA has established cooperative programs with U.S. aircraft manufacturers to facilitate this comprehensive transfer of this technology by making these advanced methodologies available to industry.This paper presents the analytical framework for predicting the onset of widespread fatigue damage. After a discussion of the results of a thorough tear-down fractographic inspection of a five-bay fuselage lap splice joint containing widespread fatigue damage, the analytical fracture mechanics requirements to predict crack initiation, fatigue crack growth, and residual strength are presented. For each of these three fracture mechanics scales, example calculations will be compared to the results of experimental verification tests.FRACTOGRAPHY OF WIDESPREAD FATIGUE DAMAGE (WFD) IN ASTRUCTURAL FATIGUE TEST ARTICLEValid analytical methodology to predict the onset of widespread fatigue damage in fuselage structure must be based on actual observations of the physical behavior of crack initiation, crack growth, and fracture. The NASA methodology is based largely on the results of teardown fractographic examinations of aircraft fuselage components. A large section of a fuselage containing a longitudinal lap splice joint extending for five bays was provided to NASA by an aircraft manufacturer after conducting a full-scale fatigue test [1]. A photograph of the panel along with a schematic is shown in Figure 1. The fatigue test was terminated after reaching the number of fuselage pressurization cycles that equaled approximately three times the original economic design life goal of the aircraft established by the manufacturer. This section of the fuselage was selected because visual inspections made during the test had detected the growth of fatigue cracks extending from adjacent rivets which eventually linked up to form a long crack that extended completely across the bay. Further visual examinations of this section of the fuselage after completing the full scale fatigue test suggested that this section contained widespread fatigue damage. All rivet holes in each of the five bays of the panel were microscopically examined for fatigue cracks. The results of this examination form the physical basis for the analytical methodology develop by NASA to predict the onset of widespread fatigue damage.There were three principal objectives of the fractographic examination of the fuselage panel. The first objective was to characterize widespread fatigue damage in a fuselage splice joint by assembling a database on the initiation and growth of fatigue cracks from rivets, including identifying the initiation mechanisms. The second objective was to provide a basis for comparing the crack growth behavior simulated in laboratory test specimens to the real behavior of an actual aircraft component. The third objective was to serve as a benchmark to verify the predictive capability of the fatigue crack growth portions of the widespread fatigue damage analytical methodology. This latter objective was achievable because the loading history of the full-scale fatigue test article was fully documented. Also, periodic underloads during the fatigue test were used to establish marker bands on the fatigue crack surfaces.Achieving the above three objectives resulted in the development of a very large database. The contents of this database include:•maps of cracks as a function of rivet locations in five consecutive bays;•documentation of crack growth shapes and dimensions;•identification of the crack initiation location and the initiating mechanisms such as high local stress, fretting, and manufacturing defects;•analysis of fatigue marker bands;•correlation between cycles and crack growth behavior; and•indications of out-of-plane displacements and mixed-mode fracture behavior.In addition to the extensive database assembled from the teardown fractographic examinations of the panel, sections of other retired aircraft and full-scale fatigue articles have also been examined to insure that the analytical methodology under development is sufficiently comprehensive to represent all fuselage assembly practices and design details.Several general conclusions are obvious from the database. First, fatigue cracks were present at virtually every rivet hole in the top row of rivets. The cracks ranged in size from about 50 microns to several inches. Crack initiation mechanisms included high local stresses, fretting along mating surfaces, and manufacturing defects created during the riveting process. The cracking behavior in each bay was similar and the results of the fatigue marker bands were relatively independent of rivet hole location. An example of small cracks found in the panel is shown in Figure 2. A small crack initiating due to high local stresses within the rivet countersunk hole is shown in Figure 2 (a). The accompanying schematic shows the location of the crack in the outer skin of the lap splice joint. An example of a small crack initiating due to fretting is shown in Figure 2 (b) along with a schematic showing the interface where the fretting occurred. Examples of long cracks found at rivet holes are shown in Figure 3. Figure 3 (a, b, and c) shows a crack that initiated due to high local stresses and Figure 3 (d, e, and f) shows a crack in a different rivet hole that initiated by fretting. The higher magnifications of the cracks shown in Figure 3 (c and f) help to identify the location where the crack initiated and the initiation mechanism. Referring to Figure 3 (b), it is seen that the crack has grown completely through the thickness of the outer skin and has extended a considerable distance beyond the head of the rivet. Likewise, the crack shown in Figure 3 (e) has also extended a considerable distance from the head of the rivet but has not brokenthrough the outer surface of the skin. In both cases, the crack front is curved and indicates the existence of significant bending stresses across the lap splice joint. Figure 4 shows a large crack that has been formed by the link up of the small fatigue cracks that developed at adjacent rivet holes. As can be seen in the photograph, the crack extended into the tear strap region, changed crack growth directions, and grew into a rivet hole in the tear strap. The surfaces of the individual fatigue cracks between the rivets were clearly identifiable in the fractographic examination of the long crack surface. Close examination revealed several cracks that initiated due to high local stresses and other cracks that initiated due to fretting. However, the length of all of the fatigue cracks at link up were approximately the same. This observation suggests that the long crack behavior is somewhat independent of the initiating mechanism. Furthermore, this observation and the quantitative data obtained from the marker band analyses strongly suggest that the fatigue behavior of the long cracks is deterministic and predictable.ANALYTICAL FRAMEWORK FOR THE METHODOLOGY TO PREDICT THEONSET OF WIDESPREAD FATIGUE DAMAGEIt is obvious from the above described fractographic examinations that the computational capability required to predict analytically the onset of widespread fatigue damage must be able to represent a wide range of crack sizes from the material (microscale) level to the global structural-scale level. These studies indicate that the fatigue crack behavior in aircraft structure can be represented conveniently by the following three analysis scales: small three-dimensional crack geometries at the microscale level (Scale I: Crack Initiation); through-the-thickness two-dimensional crack geometries at the local structural level (Scale II: Fatigue Crack Growth); and long cracks at the global structural level (Scale III: Residual Strength). The computational requirements for each of these three analysis scales are described in the following paragraphs.Scale I: Crack InitiationThe first analysis scale and corresponding computational capability represents the fracture mechanics of small cracks that exhibit three-dimensional crack-growth behavior. The existence and growth of these small cracks do not affect the global structural deformation states or internal load distributions. Examples of these cracks are surface and corner cracks that initiate at the edges of plates or at holes.Criterion for Crack Initiation Based on Small Crack BehaviorSmall fatigue cracks in some materials grow faster and at lower stress-intensity factor levels than is predicted from large-crack data which exhibits an apparent threshold for crackgrowth [2]. The initiation and growth of these small cracks are affected by metallurgical features such as inclusion particles and grain-boundary interactions [3]. Typical large-crack results are obtained from tests with cracks greater than about 2 mm in length. The large-crack threshold is usually obtained from load-reduction tests. Some tests and analyses have shown that the development of the threshold may be caused by an increase in crack-closure behavior as the load is reduced. Small cracks that initiate at inclusion particles, voids, or weak grains do not have any prior plastic deformation to develop crack closure. If a small crack is fully open, then the stress-intensity factor range is fully effective and the crack growth rate for the small crack is faster than the rate exhibited by the large-crack data and at a lower stress-intensity factor range.The concept of crack closure to explain crack growth acceleration and retardation was pioneered at NASA Langley almost two decades ago [4]. The closure concept is based on the postulate that the wake of plastically deformed material behind an advancing crack front may prevent the crack from being fully open during the complete loading cycle. Therefore, only part of the load cycle is effective in growing the crack. The crack closure concept has also been successfully used to explain the small-crack phenomenon exhibited by many aluminum alloys. The successful coupling of the closure methodology with the small-crack growth rate data base has resulted in a total life prediction methodology which treats initiation by predicting the growth of micron size cracks initiating at inclusion particles in the subgrain boundary microstructure [5].Computational Methodology for Predicting Crack Initiation in Riveted Structure Stress-intensity factor solutions are typically obtained from computational procedures such as the finite element analysis method. The ZIP3D computer code [6] has been developed to model three-dimensional crack configurations and to calculate the corresponding stress-intensity factors. This finite element analysis code uses an eight-node element and can be used to analyze stationary and growing cracks under cyclic elastic-plastic conditions, including the effects of crack closure. The FRANC3D code [7] also has solid modeling capabilities for three-dimensional geometries based on the boundary element method. For those crack configurations and general loading conditions that may occur for various structural components, weight-function solutions are being developed from the numerical results of parametric studies. These weight-function equations are particularly useful because the stress intensity factor solutions can be obtained from a stress analysis of the uncracked structure. Stress intensity factor solutions are currently being generated for cracks that initiate at countersunk rivet holes. Loading conditions include interference-fit stresses, clamp-up stresses, and loads transferred through a rivet. These stress-intensity factor solutions may then be used as input data for the FASTRAN II code [8] to predict fatigue crack growth. The FASTRAN II code is based on the mechanics of plasticity-induced crack closure. The effects of prior loading history on fatigue behavior, such as crack growth retardation and acceleration, are computed on a cycle-by-cycle basis. The code will predict the growth of cracks exhibiting the “small-crack effect” as well as two- and three-dimensional cracks exhibiting the classical Paris law crack growth behavior. The code hasbeen shown to be especially effective for predicting fatigue crack growth behavior in structures subjected to aircraft spectrum loads. The ZIP3D, FRANC3D, and FASTRAN II codes operate efficiently on engineering workstations, and FASTRAN II also operates on personal computers.Experimental Verification of Crack Initiation MethodologyThe small-crack effect and crack-closure analysis model, FASTRAN II, were used to calculate the total fatigue life (S-N) behavior of single-edge notched (SENT) specimens under constant amplitude and spectrum loads using an initial defect size based on microstructural data at initiation sites. Predicted results for aluminum alloy 2024-T3 were made using an initial semicircular crack size, 0.00024″ (6 microns), that had an equal area to the average inclusion-particle sizes that were experimentally observed to initiate cracks [3]. Comparisons of experimental and predicted fatigue lives of the SENT specimens under the TWIST [10], FALSTAFF [11], and Gaussian [12] load sequences are shown in Figure 5. The specimens were cycled until a crack, length 2a, had grown across the full thickness, B. The predicted lives are in very good agreement with the test data.Scale II: Fatigue Crack GrowthThe second analysis scale and corresponding computational capability represent the fracture mechanics of fatigue cracks that extend through the thickness of a skin or stiffener and are no longer three-dimensional in their crack-growth behavior.Crack Closure Concept for Fatigue Crack GrowthAs discussed in the previous section, the plasticity-induced crack closure model has been shown to be quite accurate in predicting the fatigue crack growth in aluminum alloys for a number of basic crack configurations for both constant amplitude and spectrum loads. The closure model is very accurate for a full range of R ratios and spike overload conditions provided the crack growth rate data are correlated with the effective stress-intensity factor range.Computational Methodology for Fatigue Crack Growth in Riveted StructureTwo-dimensional analyses are typically quite adequate for predicting crack growth. However, accurate modeling of structural details is required to provide high-fidelity results for the local stresses in a structure so that the fracture mechanics calculations will be accurate. The FRANC2D finite element analysis code [13] has been developed for the analysis of two-dimensional planar structures, and the STAGS (STructural Analysis ofGeneral Shells) nonlinear shell analysis code [14] has been developed for general shell structures. The FRANC2D code, developed by Cornell University, is a user-friendly engineering analysis code with pre- and postprocessing capabilities especially developed for fracture mechanics problems. The code operates on UNIX-based engineering workstations with X-Windows graphics and is interactive and menu driven. A unique capability of the code is the ability to predict non-self-similar crack growth behavior. An automatic adaptive remeshing capability allows an engineer to obtain a history of the stress-intensity factors for any number of cracks in the structure and for any arbitrary crack growth trajectory. The STAGS finite element code, developed by Lockheed Palo Alto Research Laboratory, provides the capability to model any general shell structure and has both geometric and material nonlinear analysis capabilities. STAGS is particularly well suited for analyzing shells that have structural features such as frames, stiffeners, and cutouts. The code uses the Riks arc-length projection method and computes large displacements and rotations at the element level. The code has been developed especially for nonlinear stability and strength analyses. Both FRANC2D and STAGS can calculate the history of the stress intensity factors for a growing crack that are compatible with FASTRAN II so that fatigue crack growth analyses may be performed. Other crack growth models may also be used. STAGS and FRANC2D operate on engineering workstations and mainframe computers.The advanced durability and damage-tolerance analysis capabilities developed in the NASA Airframe Structural Integrity Program will also be implemented in the NASGRO analysis code [15]. NASGRO is a general-purpose damage tolerance analysis code being developed by NASA Johnson Space Center. The code is based on fracture mechanics principles and may be used to compute stress intensity factors, fatigue crack growth, critical crack sizes, and the limit of safe life. An extensive library of stress intensity factors may be used with NASGRO or solutions may be obtained from a boundary element analysis capability using the FADD analysis code [16]. NASGRO also has an extensive material property library which includes most aluminum alloys, titanium alloys, and steels commonly used in the aerospace industry. Fatigue crack growth may be computed from a crack-closure mechanics model or from one of several empirical models commonly used by industry. NASGRO is used extensively throughout the aerospace industry. FADD was developed at the University of Texas and uses the distributed-dislocation method to compute stress intensity factors. This approach combines a highly accurate stress intensity factor analysis with the modeling simplicity of the boundary element analysis method. FADD is also available in a stand-alone version and is currently being tested by industry at beta-site locations. NASGRO operates on engineering workstations and personal computers. FADD is also available as a stand-alone code and operates on personal computers.Experimental Verification of Fatigue Crack Growth MethodologyAs part of the experimental verification of the fatigue crack growth methodology, fatigue tests on single shear riveted lap joint specimens were conducted to measure the growth of cracks from countersunk rivets holes [17]. The specimens were made from 0.040-inch-thick Alclad 2024-T3 sheet material and 5/16″ rivets. A small EDM notch was used toinitiate the fatigue crack at the rivet hole. The EDM notch was placed in the countersunk hole before the rivet was expanded. Tension-tension fatigue tests (R=0.02) were conducted and the growth of the fatigue crack from the EDM notch was recorded throughout the test. The growth of the through-thickness cracks were recorded along the surface of the specimen using an optical microscope. Special restraints were used to prevent the large out-of-plane rotations caused by the eccentricity in the load path of the single shear specimen. The fatigue tests were terminated usually after appreciable crack growth was recorded. Several specimens were cut apart and the interference fit was estimated by measuring the size of the rivet hole after expanding the rivet relative to the original machined hole size.The FRANC2D code was used to analyze the specimen and compute the crack growth rates. The analysis included the effects of interference fit stresses, bearing load distribution in the countersunk hole, and an approximation of the stress concentration produced by the three-dimensional geometry of the countersunk hole. The experimental measurements of the interference fit expansion was used to compute the interference fit stresses. The analytical reduction of the data recorded during the fatigue tests is shown in Figure 6. The measured crack growth rates are plotted against the computed stress-intensity factor range. The open symbols are the crack growth test data from the single shear specimens. The solid line is the baseline da/dn crack growth data for aluminum alloy 2024-T3 measured from standard crack growth tests. The length of the cracks growing from the rivet in the lap specimen were measured experimentally and the FRANC2D model was used to calculate the corresponding stress-intensity factors. The coalescence of the data from the single shear lap specimen around the baseline crack growth data confirms the accuracy of the FRANC2D analysis to properly account for the complex stress state at the countersunk rivet. The gray shaded area shows the values of the crack data if the stress intensity factors were computed without including interference fit stresses. Without the interference fit, the cracks grow at a faster rate for the same applied stress. The benefit in the crack growth rate is produced by the tensile residual stresses caused by the interference which results in a reduction to the stress intensity-factor range.Scale III: Residual StrengthThe third analysis scale and corresponding computational capability represent structures with long cracks that change the internal structural load distribution that exhibit behavior strongly affected by structural details and that affect the residual strength of the structure. In addition, the fracture mechanics of ductile materials such as 2024-T3 aluminum alloy often requires an elastic-plastic stress analysis capability that predicts stable tearing and fracture. Furthermore, nonlinear geometric effects, such as crack bulging in shell structures, also significantly affect residual strength predictions. All of these complexities are present in a fuselage shell structure and must be represented in a residual-strength analysis of the fuselage.The structural analysis computer codes under development in the NASA Airframe Structural Integrity Program are being integrated into an analytical methodology for predicting the residual strength of a fuselage structure with one or more cracks. The analytical prediction of the residual strength of a complex built-up shell structure, such as a fuselage, requires the integration of a ductile fracture criterion, a fracture-mechanics analysis, and a detailed stress analysis of the structure. The crack tip opening-angle (CTOA) criterion has been experimentally verified to be a valid fracture criterion for mode I stress states in thin and moderately thick (0.5 inch thick or less) aluminum alloys. The CTOA criterion has been demonstrated to be valid for predicting the linkup of a long lead crack with small fatigue cracks ahead of the advancing lead crack. This fracture criterion has been implemented into the STAGS geometric and material nonlinear finite element-based shell analysis code to provide an integrated structural-integrity analysis methodology. The capability to model a growing crack that may extend in a non-self-similar direction has been added to the STAGS code along with an automated mesh refinement and adaptive remeshing procedure. The topological description of the growing crack is provided by the FRANC3D fracture mechanics code. The geometric nonlinear behavior of a stiffened fuselage shell is currently under study for internal pressure loads combined with fuselage body loads that produce tension, compression, and shear loads in the shell.The CTOA Fracture Criterion for Residual StrengthThe critical crack tip opening-angle (CTOA), or equivalently, the crack tip opening-displacement (CTOD), fracture criterion is a local approach to characterizing fracture. In contrast, the J-integral or J-R curve criterion is based on global deformations and has been found to be specimen and crack-size dependent for structures with large amounts of stable tearing. The constant CTOA (or CTOD) criterion has been used to predict the variations in J-R curves due to differences in crack sizes and specimen types. Therefore, a local crack tip displacement is a more fundamental fracture parameter than the J-integral representation for local strain-controlled fracture processes such as stable tearing and void coalescence.Simple plastic-zone models that are based on linear-elastic stress intensity factors can be adjusted to fit experimental data and then used to predict crack linkup for relatively simple structural geometries. While these methods predict the correct trends in crack linkup behavior, they may be difficult to apply to analyses of complex structural details that are characteristic of a fuselage structure. The CTOA criterion can be effectively implemented into a finite element analysis code provided that the code has elastic-plastic deformation and crack-growth simulation capabilities. These capabilities exist in the STAGS geometric and material nonlinear shell analysis code, but analyses of large-scale problems must currently be conducted on a high-performance mainframe computer. After thorough experimental verification of the residual strength analysis methodology, it is anticipated that the methodology can be simplified by taking advantage of appropriate engineering approximations.。
ASTM E 647
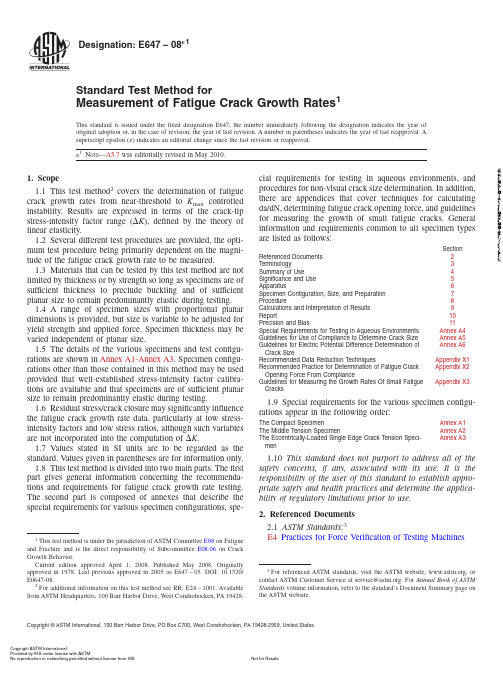
Copyright © ASTM International, 100 Barr Harbor Drive, PO Box C700, West Conshohocken, PA 19428-2959, United States.
Copyright ASTM International Provided by IHS under license with ASTM No reproduction or networking permitted without license from IHS
Designation: E647 – 08´1
//^:^^#^~^^"~~::"^#$~@@""#:$@"""^^~^:#~~@^^$^@^^:#::$\\
Standard Test Method for Measurement of Fatigue Crack Growth Rates1
This standard is issued under the fixed designation E647; the number immediately following the designation indicates the year of original adoption or, in the case of revision, the year of last revision. A number in parentheses indicates the year of last reapproval. A superscript epsilon (´) indicates an editorial change since the last revision or reapproval.
材料科学专业英语词汇(F)_材料专业英语词汇
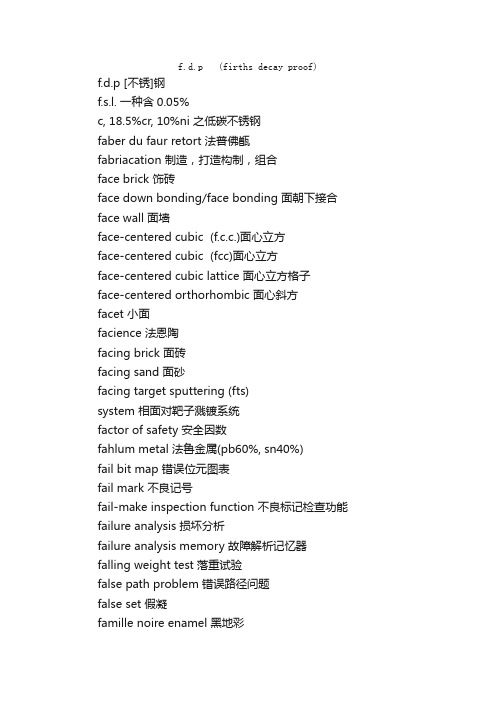
f.d.p (firths decay proof)f.d.p [不锈]钢f.s.l. 一种含0.05%c, 18.5%cr, 10%ni 之低碳不锈钢faber du faur retort 法普佛甑fabriacation 制造,打造构制,组合face brick 饰砖face down bonding/face bonding 面朝下接合face wall 面墙face-centered cubic (f.c.c.)面心立方face-centered cubic (fcc)面心立方face-centered cubic lattice 面心立方格子face-centered orthorhombic 面心斜方facet 小面facience 法恩陶facing brick 面砖facing sand 面砂facing target sputtering (fts)system 相面对靶子溅镀系统factor of safety 安全因数fahlum metal 法鲁金属(pb60%, sn40%)fail bit map 错误位元图表fail mark 不良记号fail-make inspection function 不良标记检查功能failure analysis 损坏分析failure analysis memory 故障解析记忆器falling weight test 落重试验false path problem 错误路径问题false set 假凝famille noire enamel 黑地彩famille rose 胭脂红;粉彩famille verte 绿地形family box 族系箱family head 族系原头faraday effect 法拉第夜应faraday system 法拉第系统fast breeder reactor 快滋生反应,快增殖反应器fast neutron 快中子(能量超过0.1mev)fast traverse 快速通过速度fat clay 富黏土(从土木)fat sand 高黏土砂fatigue corrosion 疲劳腐蚀fatigue crack 疲劳裂痕fatigue fracture 疲劳破裂fatigue limit 疲劳限fatigue notch factor 疲劳凹痕因数fatigue test 疲劳试验fatigue test machine 疲劳试验机fatigue tester 疲劳试验机fault 缺陷fault analysis 故障分析fault circuit 故障电路fault coverage 故障概括率fault dictionary 故障辞典fault simulator 故障模拟器fayalite 铁橄榄石,铁矽酸盐faying surface1 接合面2 待接合面feal 费尔合金(25%al, 其余为fe 有良好制振性) feather combing 梳花feather marking 羽状痕迹feed gums 种籽胶feed ring 环冒口feed riser 冒口feed water 给水feeder 饲机feeder channel 饲槽feeder gate 饲料闸feeding rod 冒口搅棒feldspar 长石feldspathic glaze 长石岫feldspathoid 似长石felsophyne 矽长斑石felsparfeldspar feltmetal 金属纤维毡feran 包铝钢条fermi level 费米准位(原子)fermi surface 费米面(原子)fermium (fm, 100)镄fernichrome 费理铬(一种能封入铅玻璃内之材料,含37%fe,30%ni,25%co,8%cr)ferrari cement 佛拿里水泥ferric oxide 三氧化二铁ferrimagnetism 亚铁磁性ferrious material 铁系金属ferrite1 肥粒铁2 磁性氧化物ferrite (ceramic)1肥粒铁2磁性氧化物ferrite grain size 肥粒铁晶粒度ferrite martensite 肥粒形麻田散体ferritic cast iron 肥粒铁铸铁ferritic decarburized depth 脱炭层之肥粒铁深度ferritic stainless steel 肥粒铁不锈钢ferro boron 硼铁ferro-alloy (ferroalloy)合金铁ferro-bronge 铁青铜(8%fe, 0.7%cr, 其余为cu)ferro-chrome 铬铁ferro-electric materials 铁电材料(如batio3)ferro-manganese 锰铁ferro-molybdenum 钼铁ferro-phosphorus 磷铁ferro-silicon 矽铁ferro-titanium 钛铁ferro-tungsten 钛铁ferro-vanadium 钨铁ferrochrome-silicon 矽铬铁ferroele ctric 强电介体(从电机)ferroelectric thin film 铁电薄膜强诱电体薄膜ferrograph 磁滞仪ferrolum 包铅钢ferromagnetic 强磁的ferromagnetic domain wall 铁磁区壁ferromagnetic spinel 铁磁性尖晶石ferromagnetism 铁磁性,强磁性ferromanganese-silicon 矽锰铁ferron 福朗(含35%ni, 15%cr,其余为铁的一种抗氧化合金) ferronickel 镍铁ferrose-forric oxide 磁性氧化铁(fe3o4) ferroselenium 矽铁ferrostan 电镀马口铁(镀锡冷卷钢)ferrous alloy 铁合金ferrous metallurgy 钢铁冶金学ferrous oxide 氧化亚铁ferrox cube 软磁磁体族ferry 福里合金(一种含55%cu, 45%ni 之高电阻材料,类似康史登钢)fertile material 可育材料fery radiation pyrometer 费氏辐射高温计fetrafluoroethylene oxide 氧化四氟乙烯fettling 修整(1 铸件加工前之修整 2 炉衬之修整) fiber metal 纤维金属fiber reinforced plastice (frp)纤维加强塑胶fiber stress 纤维应力fiberfax 岩绒,金刚纤维fiberglass 玻璃纤维fibre, ceramic 陶瓷纤维fibrous falc 纤维状滑石fibrous structure 纤维组织fick's first law 菲克(扩散)第一定理fick's law 菲克[扩散]定律fick's second law 菲克(扩散)第二定理fiducial mark 基准标记field batch weight 工地盘重field control 工地控制field effect transistor (fet)场效应电晶体field-drain pipe 工地排水管figured glass 压花玻璃filberflow 纤维流纹filed programmable gate array 现场可程式闸门阵列filed programmable interconnect 现场可程式互接filler 填充料filler metal 熔填金属fillet 接合剂渗出痕迹,接合轮廓film strength 乾釉强度film test 薄膜试验filter (活性炭)过滤器filter block 泸砖filter candle 泸筒filter cke 泸饼filter cloth 滤布filter press 压滤机filtration equipment with coagulation 凝聚过滤设备fin 缝翅final energy magnet 终极能量磁铁final polishing 最後加工抛光仕上研磨final test 最後测试final wafer test 晶圆後端测试fine aggregate 细骨材fine alignment 精细对准fine gilt 薄层镀金fine grain 细晶粒,细[颗]粒fine grinder 细研机fine grinding 细磨fine pearlite 微粒波来铁fine pitch 精细节距fine powder 超微粒fine-grained steel 细晶粒钢fineness moldulus 细度模数fineness of cement 水泥细度finess number 粒度(铸砂)finger gate 指形铸口finial 屋脊饰fining 澄清finishability 饰面难易度finished steel 精炼钢finishing impression 完成型finishing machine 整面机finishing mill 完成巴机finishing roll 未巴辊finishing temperature 完成温度fink process 芬克浸铝法finkle-mchr process 芬克莫尔法finl set 终凝finyl chloride polymers 氯乙烯聚体fir brick 火砖fir resistance 耐火性fire back 垫[背]火砖fire box 火箱fire brick 耐火砖fire bridge 火坝fire clay 耐火泥fire crack 热裂fire finished 火修光fire magnesite-chrome brick 烧镁铬砖fire mark 火痕fire pillar 火柱fire polished 火焰磨光fire proofing tile 坐火瓦fire refining 火法精炼fire sand 耐火砂fire welding 固态接合,接火fire-box steel 火箱钢fire-cracking 热裂firecaly 火黏土fired chrome brick 烧铬砖fired magnesite brick 烧镁砖firestone 耐火石firing1 烧成2点火firing expansion 烧制膨胀firing range 烧制范围firing shrinkage 烧[成][收]缩firingtemperature 烧成温度first bonding 第一接合,第一压接first coat1 底漆2 第一涂层first dislocation generation 初次发生位错first stage annealing 第一段退火firsts 一级品firth hardometer 费氏硬度计firty 耐火性fish scale 鳞状破面fish-scale 鳞剥fisheyes 鱼眼(因氢脆性之裂开面)fishtail 鱼尾式fissile material 可分裂材料fission 分裂fission fragment 分裂碎片fission poison 分裂毒物fission product 分裂产物fissionable material 可分裂材料fissium 分裂碎片合金fitting 配件five points thickness variation5 点厚度变动fixed carbon 固定碳fixing block 受钉砖块fixture board 夹具基板fizeau interferometer method 菲氏干涉计法flabby cast 软铸物flake1 小片2 裂纹3 白疵flake graphite 片状石墨flake graphite cast iron 片状石墨铸铁flaking 剥落flame annealing 火焰退火flame cleaning 火焰清除法flame cutting 火焰切割flame hardening 火焰硬化flame photometer 焰度计flame plating 焰镀[法]flame scaling 焰烤锌皮法flame sensor 火焰感测器flame softening 火焰软化flame spectro fluorimetry 火焰光谱分析flame spraying 火焰喷涂flame straightening 火焰矫直flame strengthening 火焰强化flame-plating 火焰涂层flame-spraying 焰喷[法]flame-tempering 火焰回火flameless combustion 无焰燃烧flanging 摺缘flappin [of copper] 翻搅flare bed 发焰道flare test 顶进定量变形试验(与drift test 同) flaring 扩口flash 飞边,溢边flash and fire point test 闪点及燃点试验flash lamp annealer 闪光灯退火处理机flash memory e/w cycle test system 快闪记忆体删除写入周期测试系统flash point 闪点flash radiography 闪烁放射性摄影flash roasting 闪燃煆烧flash shield 溢边护罩flash wall 闪火墙flash welding 闪电熔接flash-butt welding 闪电对头熔接flask1 砂箱2 烧瓶flat bar1 扁条2 扁条钢flat collet 平面吸具flat dies 平模flat grain 平纹flat zone length 热平坦区长度flat-die forging 平模锻造,平模锻件flat-die hammer 平模鎚flat-ware 浅皿flatness 平面度flatness quality area 平面度适用区域flats 扁钢(1/8~1/4寸厚两尺以卷宽之卷钢片)flattener 矫平机具flatting 平整[法]flemish bond 法式砌法(从土木)flexibility 凸挠性flexural strength 抗弯强度flexure tests 弯曲试验flint 火石,燧石flint clay 燧石黏土flint glass 火石玻璃flint shot 燧石粒flip chip bonder 倒装片接合机flip chip bonding 侧装片接合float glass process 浮制玻板法float polishing 浮动抛光float test index 浮游试验指数floatation equipment 漂浮分离设备floater 浮舟(玻)floating agent 浮剂floating die 浮动模floating license 浮动许可证floating zone crystal growing 浮融带晶体生长法floating zone melting method 浮动区熔法floating-zone refining 浮融带纯化floc testwater test flocculant 絮凝剂flocculation 絮凝,凝聚flong 纸型(活字版铸版用)flood casting 泄注法floor brick 地砖floor molding 地模法floor planner 平面规划器floor quarryquarry tile floor tile 铺地砖flotation 浮选flow ability 流动性flow ability of sand 铸砂流动性flow blue 霁青flow brightening 亮锡法flow coating 流动施膜flow line1 加工流程2 锻造流线flow line network 流线网,流程网flow machine 致流机flow off 溢流口flow pattern defect 流体图案缺陷flow spinning 切刀旋转法flow stress 流度应力flow table 流度台flow test 流度试验flow through 溢流道flow welding 熔流熔接fluctuating stress 波动应力flue gas 烟道气fluid-energy mill 流体能磨fluidity 流体性fluidity index 流体性指数fluidized bed 流体化床fluidized sand 流动性铸砂fluidized sand molding 流动性铸砂造模法fluidized-bed coating 流体床法护膜fluon 氟罗央(ptfe 的商业名称) fluorescence 萤光fluorescence analysis 萤光分析fluorescence particle inspection 烛光颗粒检验fluorescent material 萤光材料fluorescent penetrant test 萤光透入剂试验fluorite 萤石fluorite (fluorspar)萤石fluoroscopy 萤光分析术fluorspar 萤石flush quenching 闪激卒火flux1 助熔剂2焊剂3熔剂4通量(磁电)flux factor 熔剂因数flux line 玻膏面flux-covering 熔剂覆盖fluxing 熔剂处理fly ash 飞灰flying arch 跳顶拱flying saw 随动锯机flying shear 随动剪机flying spot microscope 飞点式显微镜foam concrete 泡沫混凝土foam glass 泡沫玻璃foamed clay 起泡黏土foamed concrete 起泡混凝土foaming 起泡focal plane 焦点平面focal plane deviation 焦点平面偏差fog quenching 喷雾卒火foil 箔foil heat treatment 箔袋热处理fold 摺痕folding defect 摺叠瑕疵follow board 嵌模板fool's gold 愚人金(黄铁矿fes2) foolner process 福药法(磷酸盐处理) foot print 脚印footing 基脚foppl test 福贝耳[硬度]试验forbidden band 禁带(原子)force fill process 加压填埋空隙forced draft 强制通风forced gas convection 强制气对流fore blow 前期吹风fore hand welding 前进熔接fore hearth 前炉foreign atom 外来原子forge hammer 锻鎚forge iron 锻铁forge rolls 锻辊forge welding 锻造熔接,锻接forgeability 锻造性forged steel 锻钢forging 1锻造2锻件forging dies 锻模forging machine 锻机forging, closed-die 1闭模锻造 2 闭模forging, cold 冷模forging, flat-die 1 平模锻造 2 平模forging, hammer 鎚锻forging, hand 手工锻造forging, high-energy-rate 高能率锻造forging, high-velocity 高速锻造forging, hot 热锻forging, machine 机械锻造forging, open-die1 开模锻造2 开模锻件forging, pancake 扁圆锻件forging, press 压机锻造forging, roll 轧机锻造forging, smith 手工锻造forging, upset1 锻粗锻造2 锻粗锻件fork 铁叉form chamfering 整形去角取面formability 成形性formal verification 正式验证formatter 格式编制器forming 成形forming gas 组成气体forming, bulge 胀大成形forming, compression 压缩成形forming, contour roll 型辊成形forming, creep 潜伸成形forming, drop hammer 落鎚成形forming, electrohydraulic 电液成形forming, electromagnetic 电磁成形forming, explosive 爆炸成形forming, high-energy-rate 高能井成形forming, hydraulic 液压成形forming, magnetic pulse 电磁震动成形forming, multiple-slide 多滑块成形forming, press 压机成形forming, press-brake 折条机成形forming, radial-fraw 径拉成形forming, rubber-diaphram 橡胶膜成形forming, rubber-pad 橡胶垫成形forming, rubber-punch 橡胶冲成形forming, shrink 收缩成形forming, stretch 拉伸成形forming, three-roll 三辊成形forming, wacuum 真空成形forming, wire 线料成形forms 模板forrester flotation machine 富尔斯特浮还机forsterite 镁橄榄石forsterite (mg2sio4)矽酸镁石forward annotation 正向注解forward extrusion 顺向挤制forward spinning 顺向旋挤forward welding 前进熔接foucault current method 傅科电流法fouling 积垢(船底海藻贝壳)fouling index(fi)污浊指标(fi)fouling resistance 防垢性(铅底) foundation 基础founder 铸工founding 铸造foundry1 铸造2铸造场foundry coke 铸焦foundry defects 铸疵foundry ladle 铸造浇斗,铸造盛通foundry losses 铸造损耗foundry pig 铸造生铁foundry pig iron 铸造用生铁foundry returns 回炉料foundry sand 铸砂foundry type metal 手植活字合金four (point)probe method 四(点)探针法four-high mill 四辊巴机,四重式轧机four-way type 四方向方式fourier transform infrared spectroscopy 傅立叶转换红外光谱学four(point)probe method 四(点)探针法fractional crystallization 分段结晶fractography 断口型像学fracture mechanics 破断力学,破坏力学fracture stress 破断应力fracture surface 破断面fracture test 破断试验fracture toughness 破裂韧性fracture transition 脆断转移[温度]frame filter press 框式压泸机frame memory 视帧记忆器;图框记忆器frame work structure 构架结构framework 架构francium (fr, 87)金法frand-read source 法一瑞式[差排]源frank partial dislocation 法兰克部份差排frary metal 法拉钡合金(一种铅钨钡合金)free carbon 游离碳free cementite 游离雪明碳铁free cutting brass 易切黄铜free cutting bronze 易切青铜free cutting steel 易切钢free electron 自由电子free energy 自由能free ferrite 游离肥料铁free lime 游离石灰free water 游离水份free-cutting metal 易切金属free-flow bender 开放弯曲机freeze-casting 冻铸法freezing 冷凝,冻结凝固freezing and thawing test 冻结融解试验freezing cuve 冷凝曲线freezing method 冷凝法freezing point1 凝固点; 2 冰点freezing range 凝固范围french chalk 法兰西白呈(即滑石3mgo4sio2、h2o)french metal 法兰西金属(一种精炼锑)french process 法兰西法(一种提锌法)frenkel defect 佛兰克缺陷(晶格缺陷)fresh water 淡水fretling corrosion[ 紧压]磨蚀,移擦腐蚀friction element 摩擦元件friction oxidation 摩擦氧化friction pressfriction-screw press friction sawing 摩擦锯切friction screw press 摩擦螺杆压机friction welding 摩擦熔接frictional loss 摩擦损耗frifluoroacetylnitrile 亚硝酸三氟乙醯fringe 晕纹frit 熔块;玻料fritted glass ware 玻釉玻璃器fritted glaze 熟釉;玻料釉fritted porcelain 玻料瓷fritting1 烧结(同sintering) 2 上釉料frizzling 绉缩(陶)frog ring 辙叉环fromont test 傅瑞孟[冲击]试验front end design 前端设计front side reference method 前侧基准法front slagging 前渣法frontier alloy 佛兰提合金(一种铝锌合金) frost glass 磨砂玻璃froth flotation 泡漆浮选fry's reagent 傅蕊族剂fuel cell 燃料电池fuel cell stack 燃料电池堆fuel cycle 燃料循环fuel element 燃料元件fuel fabrication 燃料构制fuel oil 燃料油fuel ratio 燃料比fuel reprocessing 燃料再处理fugacity (fugasity)1 挥发度 2 挥发性full annealing 完主退火full automatic growing furnace 全自动成长炉full cutting 全切割full hardening 完全硬化full insert 整体嵌件full mold process 全模法full site 全体区分地段full-killed steel 全静钢full-mould casting process 金模铸造法fuller 半圆型鎚fuller earth 漂白土fuller's ideal curve 富乐理想曲线fullering 歛缝,填隙fullering bar 填隙棒fullering fmpression 歛缝型fully-killed steel 全静钢fulminates 雷酸盐(不稳定易爆材料)fume 烟气function test 功能测试functional ceramics 功能陶瓷functional description language 功能记述语言functional schematic editor 功能检图编辑器fundamental vector furnace 基本向量炉fundation translation vector 基本平移向量funerary ware 明器furnace angle 炉体倾斜角furnace annealer 电热炉退火处理机furnace lift travel 炉移动行程furnace lining 炉衬furnace tube cleaning equipment 炉心管洗涤设备furnace, baking 烘焙炉furnace, batch [type] 批式炉furnace, bell-type 钟罩式炉furnace, bogie hearth 钟罩式炉,拖车式炉furnace, box 箱式炉furnace, car bottom 车底式炉furnace, chain type 链式炉furnace, continuous 连续式炉furnace, continuous-strand 连绩回式炉furnace, conveyor (conveyer)输送带炉furnace, direct arc 直接电弧炉furnace, high frequency 高周波电炉furnace, holding 保温炉furnace, indirect arc 间接电狐炉furnace, lift off cover 离盖式炉furnace, overhead conveyer 高吊输送炉furnace, pit 坑式炉furnace, preheating 预热炉furnace, pusher 推式炉furnace, remelting 再泰炉furnace, roller hearth 滚子炉furnace, rotary-hearth 转膛炉furnace, screw feed 螺旋送料炉furnace, slot-type 槽式炉furnace, tunnel 隧道炉furnace, walking beam 动梁炉fuse data 熔丝资料fuse link 链丝熔丝fuse-quench process 熔化急冷法fused alumina 熔凝铝氧fused quartz 熔凝石英fused sand 熔砂fused silica 熔矽石fused zone 泰融带fused-cast refractory 熔铸耐火材料fused-salt electrolysis 泰盐电解fused-silica frick 熔凝矽砖fused-silica refractory 泰凝矽质耐火材料fusible alloy 易熔合金fusible pluger 易熔塞fusing pyrometer 融点高温计fusion1 熔化,熔解 2 熔合(核子)fusion casting 熔铸法fusion of packing materials 填料熔合fusion penetration 熔融穿入fusion point 熔融点fusion range 熔融区fusion sawing 熔融锯fusion temperature 熔融温度fusion welding 熔融泰接fusion-flow test 熔流试验(搪瓷) fuzzy texture 疏松结构(搪瓷)。
材料失效分析(英文文献)
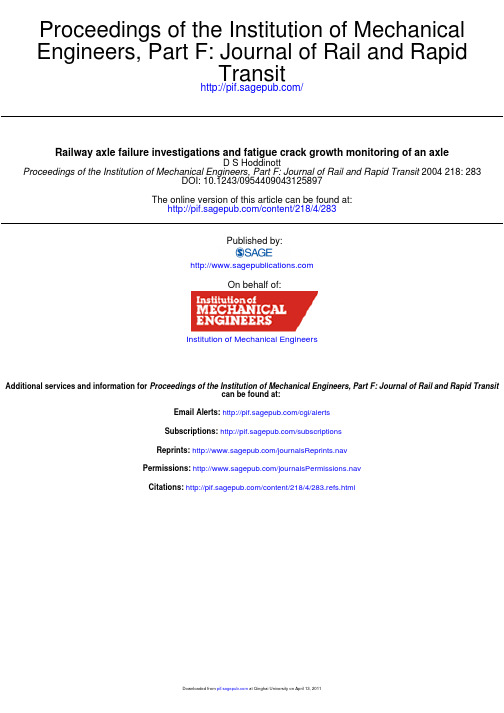
/Transit Engineers, Part F: Journal of Rail and RapidProceedings of the Institution of Mechanical/content/218/4/283The online version of this article can be found at:DOI: 10.1243/09544090431258972004 218: 283Proceedings of the Institution of Mechanical Engineers, Part F: Journal of Rail and Rapid Transit D S HoddinottRailway axle failure investigations and fatigue crack growth monitoring of an axlePublished by: On behalf of:Institution of Mechanical Engineers can be found at:Proceedings of the Institution of Mechanical Engineers, Part F: Journal of Rail and Rapid TransitAdditional services and information for/cgi/alerts Email Alerts:/subscriptions Subscriptions: /journalsReprints.nav Reprints:/journalsPermissions.nav Permissions:/content/218/4/283.refs.html Citations:SPECIAL ISSUE PAPER Railway axle failure investigations and fatigue crack growth monitoring of an axleD S HoddinottHM Railway Inspectorate,Health and Safety Executive,Rose Court,2Southwark Bridge,London SE19HS,UK E-mail:dudley.hoddinott@Abstract:Railway axles have typically been designed for an infinite life,with a normal maximumservice life of40years.However,some axles have failed in service.This paper describesfive axles thathave failed in service and the consequences of the failures,and suggests causes of the failures.Mosthad resulted from the slow growth of fatigue cracks across the axles.Electrical arcing,corrosion andstress corrosion cracking had initiated the fatigue cracks.An axle containing small cracks has beenreturned to service and the crack sizes regularly monitored.It is concluded that more information onaxles is required and it is recommended that information on the loads,stresses and materials datashould be obtained so that guidance for the design of axles can be updated.It is also recommendedthat earth return brush seats should be regularly tested for cracks and all axles regularly visuallyinspected for corrosion.Keywords:railway,axle,fracture,fatigue,corrosion1INTRODUCTIONRailway axles are designed,manufactured and main-tained so that they should not fail in service.Non-destructive testing(NDT)schedules are based on fracture mechanics evaluations and are set so that any cracks,too small to be identified by NDT methods,do not grow so large that the axle fractures before the next NDT examination at overhaul.Consequently,failures in service are few,but they are all potentially very serious. During the period from1996to2003there have been five mid-span failures of axles on vehicles in service in the United Kingdom,of which four axles fractured completely into two pieces.There were two derailments, at Rickerscote and Bennerley Junction,with one death at Rickerscote and several injuries.Thefive axle failures are described in section2with photographs of the fracture faces.Other photographs are presented in section3showing small cracks found in axles following the Bennerley Junction derailment,and the in-service monitoring of cracks in an axle is described.The main issues associated with axles and the information required to improve the design and operation of axles are listed in section4.The fractures and cracks described have all occurred in the mid-span section of axles at or near the centre of the axle.These are serious and can cause the derailment of the vehicle.Non-mid-span axle fractures and cracks are normally caused by axle bearing problems and are not considered here.2FRACTURE OF RAILWAY AXLESThefive mid-span failures of axles on vehicles that failed in service are described in this section,with photographs of the surfaces of the axles and the fracture faces. Fortunately,these failures were all one-off events that occurred over a period of7years and are not failures from exactly the same cause.Axles are designed for an infinite life with an effective working life of up to40 years.Proposals are made for the reasons for the premature axle failures in service.Table1gives the locations and dates of the axle failures,lists additional information on the axles,where available,and adds the causes of the failures.2.1Fracture of an axle on a freight train at Rickerscote The accident at Rickerscote,Stafford,occurred on8 March1996and is described in reference[1].There was a complete fracture of an axle and consequent derail-ment of a two-axle freight wagon containing liquid carbon dioxide.Unfortunately the derailed wagons blocked the adjacent line and were run into by aThe MS was received on12May2004and was accepted after revisionfor publication on1September2004.283 F01704#IMechE2004Proc.Instn Mech.Engrs Vol.218Part F:J.Rail and Rapid Transittravelling post office train going in the opposite direction.One person was killed and there were10 injuries.The fractured axle is shown in Fig.1.The axle was manufactured in1970and satisfied the specifica-tions in place at that time.Since its last ultrasonic test the axle had only travelled110000km.The accident had destroyed any information on crack initiation,but examination of the axle did reveal a small amount of corrosion,mostly only about0.03mm deep,with one corrosion pit0.05mm deep.It is likely that a fatigue crack initiated at a corrosion pit near the centre of the axle where paint hadflaked off the surface.The corrosion pitting increased the stress locally in the axle to initiate a fatigue crack.The crack then grew and the axlefinally broke into two pieces. This derailed the vehicle and caused the accident. Following the accident at Rickerscote a railway industry committee was set up that rationalized and improved the standard for the design,use,repair,overhaul and testing of railway wheelsets[2].2.2Fracture of an axle at Shields Junction,Scotland The mid-span fracture of an axle on a salt hopper wagon occurred on29January1998at Shields Junction, Scotland.The fracture was exactly at the mid-point of the axle.The fracture surface(Fig.2)shows that crack initiation occurred at two or more locations,with two large fatigue cracks growing on parallel planes until fracture occurred.The exact points of initiation were destroyed in the plete ductile fracture occurred when only about15per cent of the cross-sectional area of the axle remained unbroken,as shownTable1Information on axle failures(N/A,not available)Location of axle failure Date offailure VehicleAxle load(tonnes)AxlenumberYear axlemadeAxlemid-spandiameter(mm)Totaldistancetravelled(km)Distancefrom lastinspection(km)Cause of failure1.Rickerscote,Stafford 8March1996TTB2-axle pedestalsuspension tankwagon23AB102561970171N/A110000Fatigue crackingfrom corrosion pit2.Shields Junction,Scotland 29January1998PGA2-axle pedestalsuspension salthopper wagon2539N/A175N/A N/A Stress corrosionfatigue cracking3.BennerleyJunction,Nottingham 21June2002HFA MGR2-axlecoal hopperwagon2372485019681711600000168000Fatigue crackingfrom rust patch4.Luton station25July2002Class319dualvoltage(25kV a.c.overhead and750V d.c.thirdrail)EMU 12.5M168050SF19861873200000112000Fatigue crackingfrom electric arcdamage on raisedearth return brush seat5.Basingstoke17December2002Class442(750V d.c.third rail)EMU 13.53880011N/A192N/A N/A Extensive damagefrom electric arcingon raised earthreturn brushseat Fig.1Broken axle from the freight wagon involved in the accident at RickerscoteD S HODDINOTT284Proc.Instn Mech.Engrs Vol.218Part F:J.Rail and Rapid Transit F01704#IMechE2004in Fig.2.The axle contained multiple surface cracks,as shown in Fig.3,which have been highlighted by the magnetic particle inspection method.The axle was sectioned and the deepest non-fractured crack found measured 12mm from the surface into the body of the axle.The investigation concluded that the damaged bitu-men coating on the axle allowed salt solution to come into contact and remain trapped around the surface ofthe axle.The high mechanical fatigue stresses in the centre portion of the axle,combined with the highly corrosive liquid on the axle surface,produced stress corrosion fatigue cracking,as shown by the multiple cracking in Fig.3.Several cracks grew to produce the final fracture surface shown in Fig.2.Stress corrosion cracking can occur when a material is stressed from an applied load while simultaneously and continuously in contact with a corrosive fluid.The stress corrosion fatigue cracking of axles is more severe than either fatigue loading or corrosion acting separately on the axle and,as well as leading to potential failure in service,can otherwise shorten the overall life of the axle (see reference [3]).On this wagon,the bitumen coating protecting the axle became damaged and trapped salt solution,thus shortening its life.As a result of this accident and the accident at Rickerscote,maintenance procedures have been improved and axles are inspected for corrosion and magnetic particle inspections are conducted regularly in order to identify any small surface cracks [2].2.3Fracture of an axle and derailment at Bennerley JunctionAn axle on a wagon in a merry-go-round train (an MGR train carries coal between a pit or dock to a power station)fractured near Bennerley Junction,Nottingham,on 21June 2002.Several of the two-axle coal-carrying wagons were derailed and damaged the track,but there were no injuries.The fractured axle,number 724850,was tapered with a minimum diameter at mid-spanofFig.2Fracture surface of the broken axle involved in the accident at ShieldsJunctionFig.3Multiple cracks on the surface of the axle involved inthe accident at Shields JunctionRAILWAY AXLE FAILURE INVESTIGATIONS AND FATIGUE CRACK GROWTH MONITORING 285F01704#IMechE 2004Proc.Instn Mech.Engrs Vol.218Part F:J.Rail and Rapid Transit171mm where the fracture occurred.The maximum diameter of the axle adjacent to the wheels was 189mm.The fracture face is shown in Fig.4.About 120mm of the fracture face across the axle was covered with fine,parallel lines (beach marks),indicating that cracking occurred during a period of slow fatigue crack growth.The final 50mm across the fracture face shows that ductile failure occurred when about 25per cent of the cross-sectional area of the axle remained unbroken,as shown in Fig.4by the bottom part of the fracture surface.Then,the axle finally bent and broke into two pieces.The corners of the fracture faces were damaged during the derailment;this destroyed the point of initiation of the fatigue crack.The ductile final fracture is shown in Fig.4by the rough surface finish and the absence of any facets on the fracture surface.This axle had been manufactured in 1968and since then had travelled about 1.6million km.The axle had been tested using magnetic particle inspection (MPI)in 1998when it had been fitted with a new set of wheels.In the following four years the axle had only travelled 168000km.Similar axles can travel over 1.6million km in four years.It has been suggested that a small initiat-ing crack was present but had not been identified during the MPI test in 1998.Axles are prepared for MPI testing by grit blasting.It is possible that small patches of rust that had not been removed by the grit blasting had trapped the fluorescent magnetic ink so that the crack underneath the rust could not be identified.Work is being carried out by the owner of the vehicle to improve the whole MPI axle testing procedure,initially by takinginto account the human factor aspects of the repetitive testing of axles.Job rotation and enrichment to maintain vigilance is being considered,with inspectors taking breaks if their concentration is reducing and axles with known cracks being introduced into the stream of axles to be tested.In the longer term automation of the MPI procedure is being investigated.If this work is successful the improved techniques will be implemented more widely.2.4Fracture of an axle from electrical arcing on a class 319electric multiple unitAfter leaving Luton station on 25July 2002there was a bang and smoke was seen coming from a bogie on a class 319electric multiple unit (EMU).The driver reported power problems and terminated the passenger service.He suspected that a traction motor had burnt out.However,when the vehicle was inspected at the depot it was found that the driving axle number M168050SF had fractured and broken into two pieces.A derailment had been prevented by the suspension tube surrounding the axle constraining the two ends of the axle.In Fig.5the axle is shown resting in the suspension tube with the fracture across the axle in the middle of the photograph.The failure occurred as a result of a fatigue crack with the fracture surface showing beach marks typical of a fatigue failure.The shiny central area of the axle on either side of the fracture in Fig.5shows the raised earth returnbrushFig.4Fracture surface of axle number 724850from the MGR wagon involved in the accident at BennerleyJunctionD S HODDINOTT286Proc.Instn Mech.Engrs Vol.218Part F:J.Rail and Rapid TransitF01704#IMechE 2004seat.A bronze/graphite brush is sprung loaded on to the earth return brush seat.This forms part of the electrical circuit to conduct the traction motor current to the running rails (i.e.to earth).Normally the maximum current is 300–500A.Power can be supplied either from a 25kV a.c.overhead or 750V d.c.third rail electrical supply.Figure 6is a photomicrograph of a section of the axle close to where the fatigue crack initiated,showing a thin surface layer of copper (0.05mm thick)and a shallow heat-affected zone (0.6mm deep)produced by arcing.Martensite was present in the heat-affected zone,indicating that these areas had been subjected to a temperature in the region of 9508C.The martensite contained three fine cracks.In electric circuits martensite is commonly associated with arc damage.Cracks in the martensite in the heat-affected zone had probably initiated the fatigue crack that eventually fractured the axle.Axle number M168050SF had been manufactured in 1986and was estimated to have travelled about 3.2million km to the time of failure.AninvestigationFig.5The fracture through the raised earth return brush seat of axle number M168050SF on a class 319EMUFig.6A section through axle number M168050SF on a class 319EMU showing a 0.05mm thick surface layer of copper and a heat-affected zone 0.6mm thick containing martensiteRAILWAY AXLE FAILURE INVESTIGATIONS AND FATIGUE CRACK GROWTH MONITORING 287F01704#IMechE 2004Proc.Instn Mech.Engrs Vol.218Part F:J.Rail and Rapid Transitconcluded that a fault in the railway track and faults in the earth return circuits on the other three axles on the vehicle could have caused the arcing,with a current of up to about7000A.This is a commonly used earth return track arrangement and is used on25classes of EMU and electric locomotives.New NDT procedures have been developed specifically to identify cracks in the raised earth return brush seats.Eddy current methods, developed to identify cracks in the raised seats using probes directly applied to the seat,have been used on all vehicles having axles with earth return brush seats.A longer-term solution has also been developed for use at major overhauls.This is a new ultrasonic axle testing (UAT)technique using an angled probe applied to one end of the axle.This method avoids the need to remove the brush holder assembly or dismantle the axle suspension tube.The best technique can reliably detect a2mmfine saw cut,equivalent to a5mm deep crack,in the seat.Fracture mechanics techniques have shown that it is acceptable to carry out this test every200000km. These inspection procedures should identify any cracks in earth return brush seats to help prevent any further axle failures from this cause.2.5Damage to the earth return brush track on the axleof a class442EMUSmoke was reported to be inside the motor vehicle of a class442EMU near Basingstoke on17December2002. It was assumed that the smoke was coming from a faulty air conditioning unit;this was isolated and the unit completed its passenger-carrying journey.At a subse-quent examination in a depot it was discovered that there was extensive electrical damage to all the earth return brush holder assemblies on the axles.The source of smoke was traced to overheating of the earth return brush equipment on all four axles on the motor vehicle. There was extensive damage to the two bronze/graphite brushes and brush holders,springs and cables on the four axles.Figure7shows typical large craters in the brush track on axle number3880011.Flatter damaged areas contained craze cracking but the surface was too rough to highlight the cracking using NDT methods.A photomicrograph of a transverse section through the earth return brush track of axle16751reveals a white surface layer of deposited bronze and copper,with copper-filled cracks penetrating a deep band of tem-pered martensite,shown in Fig.8.The longest crack found was3mm and there were many cracks2mm long. Copper had penetrated down thefine cracks that were mainly in the martensitic zone.The investigations indicated that there had been considerable arcing between the bronze/graphite brush and the axle earth return brush seat,and that this had taken place when the axle was rotating.It was found that the springs that pressed each brush on to the axles came from a batch that was weaker than specified.As a consequence the brushes bounced on the axle when the vehicle traversed track irregularities,such as switches and crossings.These vehicles are powered by a750V d.c.third-rail electrical supply.The arcing caused the surface of the steel brush seat to transform to martensite,which then cracked.Also,the copper deposited on the surface of the brush seat from the bronze/graphite brush(composition:copper,carbon as graphite,lead and tin)was melted by the arcing and rapidly diffused into the steel along the cracks and grain boundaries.Previous trials had found that theremoval Fig.7Electrical arc damage on the raised earth return brush seat on axle number3880011on a class442 EMUD S HODDINOTT288Proc.Instn Mech.Engrs Vol.218Part F:J.Rail and Rapid Transit F01704#IMechE2004of cracking when copper penetration was present was insufficient to guarantee that the axle was safe for additional service.Consequently,all the axles on this vehicle were scrapped.The widespread use of new NDT procedures,put into place following the class 319EMU axle failure reported in section 2.4,should prevent any further serious incidents occurring from damaged earth return brush seats.3FATIGUE CRACK GROWTH MONITORING OF AN AXLE IN SERVICEAfter the Bennerley derailment,described in section 2.3,approximately 8000axles on all the MGR wagons in useor available for use were tested using the eddy current method.Cracks were found in four axles.The largest crack of 130mm circumferential length and 58mm deep was found in tapered axle number E66223manufac-tured in 1964.This crack,with paint and corrosion products removed,is shown in Fig.9.The crack is at the centre of the axle where the diameter is smallest.The fatigue crack had initiated at a corrosion pit in an area where the corrosion pits appear less dense than in adjacent areas.The cracking is similar to the cracking in the axle of the wagon involved in the Rickerscote accident,described in section 2.1.The pitting increased the local stress in the axle to initiate the fatigue crack.So far there has been no satisfactory explanation for why the crack had grown through a smoother area oftheFig.8The bronze and copper surface layer and copper-filled cracks in the raised earth return brush seat on axle number 16751on class 442EMUFig.9A 130mm long fatigue crack in axle number E66223RAILWAY AXLE FAILURE INVESTIGATIONS AND FATIGUE CRACK GROWTH MONITORING 289F01704#IMechE 2004Proc.Instn Mech.Engrs Vol.218Part F:J.Rail and Rapid Transitaxle.This axle would originally have been painted,with no other treatment or protection since new.There was also corrosion on the surface of tapered axle number 751593,manufactured in 1972.The longest fatigue crack was 17mm long and 8mm deep;it is shown in Fig.10after the crack had been broken open in the laboratory.The crack had initiated at a corrosion pit.Three other very small fatigue cracks are also visible.The axle found with the smallest cracks has been returned to normal MGR coal-carrying service and the cracks have been regularly monitored.This axle number 732240manufactured in 1971had travelled 100000km since its last overhaul and approximately 1.6million km since new.The three cracks in the axle,shown in Fig.11,were between 0.35and 0.7mm deep and 3to 3.5mm long.Following a risk assessment and with no objec-tions from relevant organizations,an MGR wagonwithFig.10A 17mm long fatigue crack in axle number 751593,shown after breaking it open in thelaboratoryFig.11Three small cracks in axle number 732240before returning the axle to service on an MGR wagonD S HODDINOTT290Proc.Instn Mech.Engrs Vol.218Part F:J.Rail and Rapid TransitF01704#IMechE 2004this axle was returned to service on15April2003.This wagon is being regularly monitored and the crack sizes measured.The axle will be permanently withdrawn from service if any crack reaches5mm deep or50mm long. These crack dimensions have been chosen so that the largest crack would still be within the regime of slow crack growth and with a considerable distance remain-ing before the crack became so long that there was a risk that the axle might fracture.So far there has been no change in the measured crack dimensions.The cracks in this axle were under tape that had been wound around the axle for identification purposes at some time after this wheelset had been assembled.The tape was around the middle of the axle at its narrowest section.This is also the position of the highest stresses in the part of the axle between the wheels.It is now known that the tape absorbs water,so that the cracks are likely to have been the result of the combination of fatigue stressing and corrosion,namely stress corrosion fatigue cracking,as discussed in section 2.2and reference[3].However,the surface cracking is much less severe than the cracking shown in Fig.3, probably because the polluted rainwater on this axle was much less corrosive than the salt solution on the axle shown in Fig.3.There are no records of any other differences between this axle and other axles,and the wheels did not have any wheelflats on them.All identification tape has since been removed from axles. Before returning the axle to service the tape and paint on the surface of the axle was removed.The continuous corrosive environment has therefore been removed as the majority of days in the United Kingdom are dry. Even on days when it rains it is unusual for rain to be falling continuously for24hours.The author suggests that the centre of the axle might be wet for only about15 per cent of the time,and considerably less than100per cent of the time when the centre of the axle was continuously surrounded by wet tape.Consequently,the mechanical fatigue stressing alone appears to be insufficient to grow these small cracks,which are likely to have been initiated by stress corrosion fatigue cracking.4ISSUES ASSOCIATED WITH THE DESIGN OF RAILWAY AXLESRailway axles are safety critical components whose failure may result in derailments,deaths and injuries. The examples shown demonstrate that railway axles have cracked and failed in service from mechanical loads,electrical arcing and corrosion.The problem is that little is known about the maximum loads applied to axles in service or the range of operating environments, including electrical arcing and corrosion effects.The present design guides for axles(references[4],[5]and[6])were issued by the companies owning railway vehicles in 1996after the privatization of British Rail(BR);these guides were based on earlier BR design guides for axles. Information is required to improve axle designs and reduce the risk of axle failures.Gaps in present knowledge would befilled by answers to the following questions to improve standards for axles:1.Loading applied to axles:(a)Has the quality of the track deteriorated?(b)How does the track quality affect the loading onaxles?(c)What load spectra are applied to an axle inservice?(d)How frequent are axle accelerations of over100g?(e)What loading data are available from measure-ments of vehicles in service?(f)How significant are the effects from using axles aselectrical conductors on the overall life of anaxle?(g)How large are the effects of corrosion fromchemicals and polluted rainwater on axles?2.Stress analysis of axles:(a)How realistic is the present stress analysis ofaxles?(b)Hasfinite element analysis been used to designaxles?(c)Can high impact loading be modelled?(d)Can axles be tapered to reduce weight withoutincreasing the maximum surface stresses?(e)How is the fatigue environment analysed?3.Materials data:(a)Is realistic fatigue crack growth rate data avail-able for axles in service?(b)How accurate and realistic are the fracturemechanics calculations?(c)What material fatigue data are available fromlaboratory tests using service loadings?(d)How susceptible are axles to surface corrosionand stress corrosion cracking?(e)How can the surface protection on axles beimproved?4.Design criteria:(a)Do the design guides take into account realisticloading data?(b)Do the design guides take into account modernstress analysis methods?(c)Do the design guides take into account up-to-datematerials data?(d)How accurate is the allowance for environmentalfactors such as electrical damage,corrosionpitting,stress corrosion cracking,physicaldamage,etc.?RAILWAY AXLE FAILURE INVESTIGATIONS AND FATIGUE CRACK GROWTH MONITORING291 F01704#IMechE2004Proc.Instn Mech.Engrs Vol.218Part F:J.Rail and Rapid Transit(e)If a vehicle is involved in a derailment does thisaffect the remaining life of the axle?(f)What degree of confidence exists in the presentdesign criteria for axles?(g)Should the maximum usable life of axles still be40years?5CONCLUSIONSIn the last few years there has been a significant number of mid-span axle failures where axles have broken into two pieces and other axles have cracked in service.Some NDT methods are better than others at identifying small cracks in axles.Examination of the fracture faces has shown that fractures have resulted from the slow growth of fatigue cracks across the axles.Electrical arcing, corrosion and stress corrosion cracking have initiated fatigue cracks.Three cracks in an axle of a freight wagon in service are being monitored.6RECOMMENDATIONSThere is some concern about the number of axles that had been designed for an infinite life but have failed prematurely in service.It is recommended that the following actions are carried out:1.The most appropriate NDT method should be usedto identify cracks in each area of axles where cracks have been known to occur.2.The effect of fatigue crack initiating factors,actingindividually and in combination,should be deter-mined.rmation should be obtained on the loadingapplied to axles,stress analysis of axles,materials data and a detailed examination made of the design criteria used for the design of axles.4.The design criteria and guidance should be revised andthe redesign of railway axles should be considered.5.The earth return brush seats of electric locomotivesand electric multiple units should be regularly inspected.6.Railway axles should be regularly visually inspectedfor corrosion which,if present,should be suitably treated.REFERENCES1Railway Accident at Rickerscote.A Report of the Investiga-tion into the Derailment of a Freight Train and Subsequent Collision with a Travelling Post Office Train on8March 1996,1996(HSE Books,London).2Railway wheelsets.GM/RT2466,Rail Safety and Standards Board,2003.3Stress Corrosion Cracking,2000(National Physical Labora-tory).4Code of Practice for the Use of BS5872—Wheelsets,1996, BASS502,Issue4,Railway Companies of1996.5Design Guide for the Calculation of Stresses in Axles Driven by Axle Hung Traction Motors,1996,BASS503,Issue C, Railway Companies of1996.6Design Guide for the Calculation of Stresses in Non-driving Axles,1996,BASS504,Issue C,Railway Companies of 1996.D S HODDINOTT292Proc.Instn Mech.Engrs Vol.218Part F:J.Rail and Rapid Transit F01704#IMechE2004at Qinghai University on April 13, 2011Downloaded from。
Fatigue crack growth behaviour and life prediction for 2324-T39 and 7050-T7451 aluminium alloys
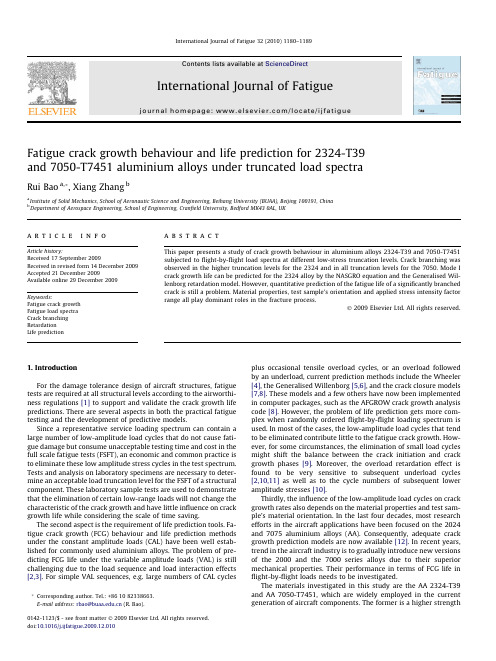
Fatigue crack growth behaviour and life prediction for 2324-T39and 7050-T7451aluminium alloys under truncated load spectraRui Bao a,*,Xiang Zhang ba Institute of Solid Mechanics,School of Aeronautic Science and Engineering,Beihang University (BUAA),Beijing 100191,China bDepartment of Aerospace Engineering,School of Engineering,Cranfield University,Bedford MK430AL,UKa r t i c l e i n f o Article history:Received 17September 2009Received in revised form 14December 2009Accepted 21December 2009Available online 29December 2009Keywords:Fatigue crack growth Fatigue load spectra Crack branching Retardation Life predictiona b s t r a c tThis paper presents a study of crack growth behaviour in aluminium alloys 2324-T39and 7050-T7451subjected to flight-by-flight load spectra at different low-stress truncation levels.Crack branching was observed in the higher truncation levels for the 2324and in all truncation levels for the 7050.Mode I crack growth life can be predicted for the 2324alloy by the NASGRO equation and the Generalised Wil-lenborg retardation model.However,quantitative prediction of the fatigue life of a significantly branched crack is still a problem.Material properties,test sample’s orientation and applied stress intensity factor range all play dominant roles in the fracture process.Ó2009Elsevier Ltd.All rights reserved.1.IntroductionFor the damage tolerance design of aircraft structures,fatigue tests are required at all structural levels according to the airworthi-ness regulations [1]to support and validate the crack growth life predictions.There are several aspects in both the practical fatigue testing and the development of predictive models.Since a representative service loading spectrum can contain a large number of low-amplitude load cycles that do not cause fati-gue damage but consume unacceptable testing time and cost in the full scale fatigue tests (FSFT),an economic and common practice is to eliminate these low amplitude stress cycles in the test spectrum.Tests and analysis on laboratory specimens are necessary to deter-mine an acceptable load truncation level for the FSFT of a structural component.These laboratory sample tests are used to demonstrate that the elimination of certain low-range loads will not change the characteristic of the crack growth and have little influence on crack growth life while considering the scale of time saving.The second aspect is the requirement of life prediction tools.Fa-tigue crack growth (FCG)behaviour and life prediction methods under the constant amplitude loads (CAL)have been well estab-lished for commonly used aluminium alloys.The problem of pre-dicting FCG life under the variable amplitude loads (VAL)is still challenging due to the load sequence and load interaction effects [2,3].For simple VAL sequences,rge numbers of CAL cyclesplus occasional tensile overload cycles,or an overload followed by an underload,current prediction methods include the Wheeler [4],the Generalised Willenborg [5,6],and the crack closure models [7,8].These models and a few others have now been implemented in computer packages,such as the AFGROW crack growth analysis code [8].However,the problem of life prediction gets more com-plex when randomly ordered flight-by-flight loading spectrum is used.In most of the cases,the low-amplitude load cycles that tend to be eliminated contribute little to the fatigue crack growth.How-ever,for some circumstances,the elimination of small load cycles might shift the balance between the crack initiation and crack growth phases [9].Moreover,the overload retardation effect is found to be very sensitive to subsequent underload cycles [2,10,11]as well as to the cycle numbers of subsequent lower amplitude stresses [10].Thirdly,the influence of the low-amplitude load cycles on crack growth rates also depends on the material properties and test sam-ple’s material orientation.In the last four decades,most research efforts in the aircraft applications have been focused on the 2024and 7075aluminium alloys (AA).Consequently,adequate crack growth prediction models are now available [12].In recent years,trend in the aircraft industry is to gradually introduce new versions of the 2000and the 7000series alloys due to their superior mechanical properties.Their performance in terms of FCG life in flight-by-flight loads needs to be investigated.The materials investigated in this study are the AA 2324-T39and AA 7050-T7451,which are widely employed in the current generation of aircraft components.The former is a higher strength0142-1123/$-see front matter Ó2009Elsevier Ltd.All rights reserved.doi:10.1016/j.ijfatigue.2009.12.010*Corresponding author.Tel.:+861082338663.E-mail address:rbao@ (R.Bao).International Journal of Fatigue 32(2010)1180–1189Contents lists available at ScienceDirectInternational Journal of Fatiguejournal homepage:w w w.e l s e v i e r.c o m/l o c a t e /i j f a t i g ueversion of AA 2024-T351and is a high-purity controlled composi-tion variant of 2024,and is mainly applied on the lower wing skin and center wing box components of new commercial transport air-craft.The fracture behaviour and crack growth behaviour of 2324have been widely investigated in recent years for better under-standing and further application of this material [13].Alloy 7050is the premier choice for aerospace applications requiring the best possible combination of strength,stress corrosion cracking (SCC)resistance and toughness.However,it is one of these highly aniso-tropic alloys;consequently,crack growth behaviour along the short transverse direction (L-S)is found to be quite different from that along the long transverse direction (L-T).Nevertheless,the L-S orientated plates have found some applications in the spar caps and stringer webs of machined integral skin–stringer panels.Pro-gress has been made in understanding the crack growth behaviour in L-S orientated AA 7050-T7451plates under CAL at different stress ratios [14,15],in which comparisons of the failure modes be-tween the L-S and T-L plates under truncated loading spectra are also presented.Small crack growth rates in AA 7050-T7451sub-jected to simple load sequences containing underloads are re-ported in [16]to generate constant amplitude crack growth data for use in life predictions.The experimental tests conducted in this study were designed to achieve two objectives:(1)to select a suitable low-load trunca-tion level for the FSFT;(2)to investigate the characteristics of crack growth behaviour under different load spectra with various trun-cation levels.The first objective was achieved and reported in [17].The purpose of this paper is to present the investigation find-ings towards the second objective,which covers the studies of crack growth behaviour under a flight-by-flight loading spectrum of a civil transport aircraft wing at different small-stress rangetruncation levels and the performance of current predictive models in spectrum loads.2.Experimental procedures 2.1.MaterialsCrack propagation tests were conducted using the middle-crack tension,M(T),specimens made of AA 2324-T39and AA 7050-T7451.The configuration and orientation of the specimens are shown in Fig.1and Table 1.The material data is found in reference [18,19].For alloy 2324,(wt.%),Si 0.1,Fe 0.12,Cu 3.8–4.4,Mn 0.3–0.9,Mg 1.2–1.8,Cr 0.10,Zn 0.25,Ti 0.15,others each 0.05,others total 0.15,aluminium remainder.For alloy 7050,(wt.%),Si 0.12,Fe 0.15,Cu 2.0–2.6,Mn 0.10,Mg 1.9–2.6,Cr 0.04,Zn 5.7–6.7,Zr 0.08–0.115,Ti 0.06,others each 0.05,others total 0.15,balance alu-minium.The mechanical properties are fully defined in [18,19]and presented in Table 2.Crack growth rate and fatigue properties are available for the L-T orientation (refer to Fig.1)for both alloys [20].A total of 66specimens were tested;for each load truncation le-vel six specimens were tested for AA 2324-T39and five specimens for AA 7050-T7451.2.2.Load spectraThe baseline load spectrum is a flight-by-flight spectrum with each load block simulating 4200flights.The gust and manoeuvres loads are represented by ten load levels flatulating around the mean load corresponding to the 1g flight condition.The flights in each load block are classified into five types,stated as A,B,C,D and E,respectively,according to the stress levels.Flight type A is the most severe loading condition occurring only once in each block,whereas flight type E is the least severe occurring 2958times in each block.The five flight types were arranged randomly within one block except that flight type A was arranged to occur near the middle and in the second half of a block.The taking-off and landing taxiing loads were taken into account by eight equiv-alent loading cycles in each flight.Fig.2a illustrates a loading seg-ment of the baseline spectrum containing the flight types A,B and E.It can be seen that the spectrum is dominated by tension loads.The baseline spectrum (S0)was filtered by removing small-stress range cycles to obtain different truncated spectra,while the taxiing loads during each flight were retained.A 9.82%trunca-Fig.1.Configuration of M(T)specimen and definition of material orientation.Nomenclature a ,a 0half crack length,half of initial crack length in middle-crack tension,M(T),specimenC ,n ,p ,q material constants in the NASGRO fatigue crack growthrate lawC f 0parameter in AFGROW crack closure model d a /d N crack growth rate E Young’s modulus f parameter in NASGRO equation taking account of thecrack closure effectK ,K max stress intensity factor (SIF),maximum SIF D K ,D K th SIF range,SIF thresholdK crit apparent fracture toughnessN number of cycles in crack growth laws and NASGRO equationN f fatigue crack growth life in terms of flightsR nominal stress intensity factor ratio (R =K min /K max =r -min /r max )SORshut-off Ratio in Willenborg retardation modelTable 1Specimen dimensions (mm)and orientation.Length (L )Width (W )Thickness (t )Half length of the saw-cut (a n )Orientation 2324-T3935098.5 4.54L-T 7050-T745135098.564L-SR.Bao,X.Zhang /International Journal of Fatigue 32(2010)1180–11891181tion level indicates that those load cycles with stress range less than9.82%of the maximum stress range in the S0were removed, while the other parts of the spectrum were kept unchanged.So no matter what the load truncation level is,the mean stress level re-mains to correspond to the1g acceleration.There arefive trunca-tion levels resulting infive different truncated load spectra,named as S1,S2,S3,S4and S5,shown in Table3.Fig.2b shows the load sequences offlight type A in S0and S5.2.3.Fatigue testingPre-cracking was accomplished under constant amplitude load of r max=90MPa,R=0.06which resulted in an initial crack length a0of about5.5mm.The FCG tests were subsequently carried out under the aforementioned six load spectra until the half crack length a was greater than24mm.All the tests were conducted using the MTS880fatigue test sys-tem.Specimens were held in a pair of100mm wide hydraulic wedge grips.An observation system consisting of a digital microscope,servo motor and raster ruler was used to record the crack tip position. Incremental crack length measurements were made on both the right and left sides of the front surface of the specimens.The fol-lowing rules were adopted when recording the crack length:(1) if it is an ideal mode I crack,which is perpendicular to the applied loads and propagating along the x-axis,the crack length is the true distance from the symmetric axis of the specimen to the crack tip, Fig.3a;(2)if the crack has deviated from the horizontal x-axis, then the crack length refers to the projected length of the crack on the x-axis,Fig.3b;(3)if the crack has branched,the recorded crack length is the projected length of the longest branch,Fig.3c.3.Prediction method3.1.Review of available crack growth prediction modelsBased on the principle of the linear elastic fracture mechanics (LEFM),FCG rates can be correlated with the stress intensity factor range D K.There are many empirical FCG laws to describe this rela-tionship.Paris law[21]is thefirst and most popular,which corre-lates crack growth rate d a/d N with only the D K.During the following decades,modifications to the Paris law have been devel-oped by taking into account of different factors.For example,the Forman[22]and Walker equations[23]were proposed to encom-pass the mean stress effect.Both the Paris and Walker equations work well for the stable crack growth stage showing good linearity in double logarithm coordinate of d a/d N vs.D K.The Forman equa-tion[22]introduces the parameter of critical stress intensity factor K c therefore can be applied in the prediction of thefinal fracture re-gime.Hartman and Schijve[24]suggested a modified form of Paris law by adding a parameter of stress intensity factor threshold which depended strongly on the alloy and the environment.The NASGRO equation[8]takes account of the influences of the mean stress,the critical and threshold SIF,and plasticity-induced crack closure by introducing several empirical constants.Most of the empirical constants in the above mentioned crack growth laws are obtained byfitting measured crack growth test data under con-stant amplitude stresses.Table2Material mechanical properties.Tensile strength(MPa)Yield strength(MPa)Elongation(%)KI C (plane strain)(MPaffiffiffiffiffimp)2324-T39475370838.5–44(t=19.05–33.02mm) 7050-T7451510441931.9(L-T),27.5(T-L)(t=25.42–50.80mm)Table3Details of the truncated loading spectra.Name of spectrum S0S1S2S3S4S5Truncation level(%)09.8211.7213.9817.1121.36Cycles eliminated in each block(%)026.5646.8762.9573.3578.581182R.Bao,X.Zhang/International Journal of Fatigue32(2010)1180–1189When variable amplitude loading is applied,crack growth behaviour shows more complicated characters.One of the most important factors that should be taken into account is the profound overload retardation effect[2,12].However,this effect is much re-duced when the tension overload is immediately followed by com-pressive underload[2,12].Furthermore,if a load history has peak loads with a long recurrence period,i.e.peak overload cycle is fol-lowed by large numbers of baseline stress cycles,it will result in large retardation in the crack growth curve,while an almost regu-lar crack growth curve may be resulted by a load history which has peak loads with a short recurrence period[10].These two factors were paid more attention in this study,since the loading spectra in this study all contain a tensile overload in theflight type A which is followed by a negative underload,and with the increase of load truncation levels,the recurrence periods between the two overload peaks become shorter and shorter.A number of crack growth models have been developed to ac-count for the load interaction effects and thereby enable predic-tions of crack growth lives.Most of the retardation models are based on either the crack tip plastic zone concept or the crack clo-sure argument.Five retardation models are available in the crack growth prediction software AFGROW;they are the Closure model [7,8],the FASTRAN model[25],the Hsu[26],the Wheeler[4]and the Willenborg models[5,6].Each retardation model has one or more user adjustable parameter(s),which are used to tune the model tofit the actual test data.Ideally,any parameter in crack retardation models should be a material constant,which is inde-pendent of other variables such as the spectrum sequence and load level.The Generalised Willenborg model is a modified version of the original Willenborg model.Physical arguments were used to account for the reduction of the overload retardation due to subse-quent underloads.The AFGROW code has adapted the treatment of the underload acceleration effect by using the Chang’s model[8,27] to adjust the overload induced yield zone size.Therefore the effect of compressive stresses after a tension overload is considered.This model is suitable to the overload/underload pattern found in the load spectra used in this study as shown in Fig.2b.The AFGROW Closure model is based on the original Elber’s crack closure concept [7]and developed by Harter et al.[8].This type of crack closure models has been very popular in both the constant and variable amplitude loads because they correlate crack growth rates with the effective stress intensity factor range,which is affected by the applied loads and crack opening displacements;both can be re-lated to the cyclic plasticity effect.The AFGROW Closure model uses a single adjustable parameter(C f0)that is determined at stress ratio R=0in order to‘‘tune”the closure model for a given material. The suggested C f0value by AFGROW for aluminium alloys is0.3[8].3.2.Crack growth and retardation laws used in this studyIn this study,FCG life predictions were accomplished by using the AFGROW computer package[20]and employing the NASGRO equation[8],Eq.(1).Since this equation takes account of the influ-ences of the mean stress,the critical and threshold SIF,and plastic-ity-induced crack closure,it usually gives more accurate predictions provided that the required material constants are available.The material constants used in the NASGRO equation for commonly used aluminium alloys,including2324-T39studied here,are provided in the database of the AFGROW package.d ad N¼C1Àf1ÀRD Kn1ÀD K thp1ÀK maxK critqð1ÞIn order tofind a suitable crack retardation model for further analyses of the truncated load spectra,attempts have been made to predict FCG life from a=5.5mm to22mm under the S0spec-trum using the Generalised Willenborg model and Closure model. The prediction results are shown in Table4.It indicates that both the No-retardation model and the Generalised Willenborg model have achieved good agreement with the test result,whereas the Closure model underestimated the life by13%using the recom-mended C f0=0.3.Prediction is found to be sensitive to the value of parameter C f0.The following crack life predictions are performed by the NASGRO equation(no retardation)and NASGRO equation plus the Generalised Willenborg model.4.Results and discussion4.1.Crack growth morphology4.1.1.AA2324-T39L-T oriented specimenIt has been observed during the experiments that cracks sub-jected to load spectra S0,S1,S2and S3are perfect mode I cracks which areflat and straight and perpendicular to the applied loads direction.However,significant crack meandering and/or branching are observed when the low-load range truncation is increased to a certain level,i.e.S4and S5,shown in Fig.4.A typical branched crack occurred under spectrum S4is illustrated in Fig.5.It is amaz-ing that the crack always tended to grow away from the centerline of the specimen rather than taking a zigzag route.The crack growth rate dropped significantly after the crack had branched.Some evidence has been found for therelationship Fig.3.Recorded crack lengths with respect to different crack morphologies.Table4Predicted FCG life using two different retardation models(spectrum S0,AA2324-T39).Model and parameter NoretardationClosure model WillenborgmodelTestresultC f0=0.3C f0=0.5SOR=3.0aFCGL(flights)12,73911,755551713,72913,495Error(%)–5.6–12.9–59.1 1.7–a SOR=3.0is recommended by AFGROW technical manual[8]for aluminiumalloys.R.Bao,X.Zhang/International Journal of Fatigue32(2010)1180–11891183between the crack growth path change and the peak stress in the flight type A.This will be discussed in Section 4.4.However,branching was not observed immediately after the maximum over-load.It can be deduced that the tension overload had introduced a few secondary cracks in the subsurface of the specimen,which were later observed at the surface of the specimen.The lead crack and the secondary cracks kept growing for a period of loading cy-cles until they were linked up,which has resulted in the observed branched crack.The appearance of the secondary cracks and the linking up process are shown in Fig.6.The final failure mode of the branched crack under tension load is almost the same as the perfect mode I crack,showing the typical characteristic of mode I crack in ductile materials,see insert of Fig.5. 4.1.2.AA 7050-T7451L-S oriented specimenCrack turning,meandering,branching and splitting (90°turn)were observed on some specimens whatever the load truncation level was applied as shown in Fig.7a.However,not all the speci-mens showed significant crack turning or branching.It is quite dif-ferent from the 2324-T39L-T specimens,in which crack branching occurred only under the spectrum S4and S5.Schubbe also ob-served crack branching and splitting in 7050-T7451L-S oriented specimens [14,15].He has pointed out that significant forward growth retardation or splitting is evident at a threshold D K valuein the range of 10–15MPa ffiffiffiffiffim p and a distinct crack arrest point where vertical growth is dominating is found when D K is between18and 20MPa ffiffiffiffiffim p [14].In this study,the D K value corresponding to the peak stress range in flight type A at initial cracklengthFig.4.Crack morphologies under different loading spectra;(a)–(f)are for the S0,S1,S2,S3,S4and S5spectrumrespectively.Fig.5.Crack turning,meandering and branching for 2324-T39(L-T)(the example shown here is under the spectrum S4of low-load range truncation level of17.11%).Fig.6.Appearance of secondary surface cracks and cracks linking up (the example shown here is under the spectrum S5of truncation level about 21.36%):(a)lead crack r ,secondary crack s (b)another secondary crack t ;(c)link-up of the two secondary cracks with the lead crack.1184R.Bao,X.Zhang /International Journal of Fatigue 32(2010)1180–1189a 0=5.5mm is already above 22MPa ffiffiffiffiffim p ,therefore the reason for observed crack branching and splitting is understood,whether or not the load spectrum was truncated.However,it should be men-tioned here that slight crack branching was also observed occa-sionally during the pre-cracking stage with fatigue crack length no more than 0.5mm from the edge of the saw-cut andD K <10MPa ffiffiffiffiffim p ,see Fig.7b.It was hard to get the normal mode I failure strength when per-forming the residual strength testing after the half crack length a had reached 24mm.Longitudinal splitting occurred parallel to the load direction,as shown in Fig.8.4.2.Crack growth livesSince the loading cycle numbers are different in different load spectra corresponding to different truncation levels,FCG life is de-fined as the number of flights,N f ,rather than the number of load cycles N .Initial crack length a 0was 5.5mm for all the specimens.Measured a vs.N f data corresponding to the S0,S2and S4load spectra are shown in Fig.9a and b for the two aluminium alloys,which indicate good linearity in the log-linear coordinate.For the clarity of illustration,measured data for spectra S1,S3and S5are omitted in these figures.They do follow the same trend and similar scatter range.Fig.9c and d present the best fitted curves of the test data with respect to all the loading spectra using exponential linear least square method,a ¼Ae BN fð2Þwhere,A and B are fitting parameters.Since a 0=5.5mm,find A =5.5.Crack growth retardation due to overload effect are not obvious in this figure for spectra S0,S1,S2,and S3.The significant retardation found in the S4and S5spectra tests was partially caused by the crack branching described in Section 4.1and partially by the removal of large numbers of load cycles at these two higher trunca-tion levels.4.3.Prediction of crack growth livesCrack growth life predictions were performed using the AF-GROW code.Predicted a –N f curves for AA 2324-T39are shown in Fig.10.The loading spectra and specimen configuration used in the prediction are the same as those used in the experimental tests.In the figures,‘‘No Retardation”means no load sequence effect was considered;‘‘Willenborg”demotes the Generalised Willenborg model.Following observations can be made:(1)For S0,S1,S2and S3,i.e.load range truncation level of 0%,9.82%,11.72%and 13.98%of the maximum load range,the predicted lives agree well with the test results.(2)Under the spectra S4and S5,17.11%and 21.36%truncation levels,measured crack growth lives are much longer than the predicted by Willenborg model.Such differences in the model and measurement cannot be related to the overload retarda-tion effect alone.The main reason is the occurrence of significant crack meandering and branching under these two loading spectra,as mentioned in Section 4.1,which slow down the lead crack growth rate significantly.This kind of crack growth retardation mechanism is very different from that due to the overload induced plastic zone effect.Crack path deviation was not observed in the tests subjected to load spectra S0,S1,S2and S3.Hence,the FCGlifeFig.7.Crack morphology of the L-S oriented 7050-T7451specimens:(a)crack branching and splitting during propagation under spectrum S0,(b)crack branching during pre-cracking under constant amplitudeloads.Fig.8.Final splitting failure of a L-S oriented 7050-T7451specimen under static residual strength test.R.Bao,X.Zhang /International Journal of Fatigue 32(2010)1180–11891185predictions are fairly accurate,even though the same tensile over-loads exist in these spectra as in the S4and S5.(3)Predicted lives obtained by the ‘‘No Retardation”model and ‘‘Willenborg”model do not differ from each other too much;both are located within the scatter band of the test data.This is mainly due to the overload pattern:an underload is immediately following the overload,see Fig.2b,reducing the retardation effect.Considering the preferred conservativeness in practical applications,NASGRO equation with-out overload retardation is applicable for such kind of load spec-trum investigated in this study with low-stress range truncation levels no higher than 14%of the maximum stress range.Since the crack growth rate data in terms of d a =d N –D K is not available for the 7050-T7451L-S orientation in the AFGROW package,FCG life prediction for this material under spectrum loads was not con-ducted.Schubbe published some d a =d N –D K crack growth data for AA 7050-T7451L-S orientation under constant amplitude loads at different stress ratios in [14].Attempts have been made to use these data and the Harter T-method to predict crack growth lives for 7050-T7451under spectrum loads.However,the d a =d N –D K curves in [14]have a characteristic divergent sinusoidal trend ofgrowth when D K >10MPa ffiffiffiffiffim p ,where the growth mode alter-nates between arrested forward growth and splitting,or arrested vertical growth and continuing forward progression.This crack growth data cannot be used in the crack growth life prediction with the AFGROW,because there is no crack growth model that can take account of such sinusoidal trend of crack growth.4.4.Crack growth rateIt has been mentioned in Section 4.3that the ‘‘No Retardation”option in AFGROW gives good FCG life predictions under the load spectra S0,S1,S2and S3.However,when the crack branching or splitting occurred under S4and S5,the observed significant crack growth retardation is not caused by the overload induced yield zone effect;hence it cannot be predicted by these retardation mod-els in AFGROW.Another point is that the crack branching was not observed immediately after the maximum stress in the block.Since it is difficult to observe the overload effect from these a –N f curves,the crack growth rate data may shed light on the effect immedi-ately after each overload.Attempts have been made to find expla-nations for the relationship between crack growth retardation and tensile overloads.Fig.11shows the measured d a /N f –N f curves for the 2324-T39L-T and 7050-T7451L-S specimens under different load truncation levels.Locations of the peak loads in the flight A are marked by the vertical lines.The effect of these peak loads on crack growth rate can be observed.These crack growth rate vs.flights curves show periodic changes in both aluminium alloys.Following obser-vations can be made:(1)The occurrences of crack retardation fol-low the maximum tension overload regularly in each load block.(2)Retardation is more significant at higher truncation levels,S4and S5,than that at lower truncation levels,S0,S1,S2and S3.(3)Crack growth retardation in 2324-T39L-T specimens is more1186R.Bao,X.Zhang /International Journal of Fatigue 32(2010)1180–1189。
In-situ-monitoring-of-fatigue-crack-growth-using-high-frequency-guided-waves

In-situ monitoring of fatigue crack growth using high frequencyguided wavesB.Masserey a,P.Fromme b,na Department of Mechanical Engineering,University of Applied Sciences,Fribourg,Switzerlandb Department of Mechanical Engineering,University College London,London WC1E7JE,UKa r t i c l e i n f oArticle history:Received27October2014Received in revised form13December2014Accepted19December2014Available online29December2014Keywords:Fatigue crackMonitoringHigh frequency guided ultrasonic wavesP/E measurementLamb wave modesa b s t r a c tThe development of fatigue cracks at fastener holes represents a common maintenance problem for aircraft.High frequency guided ultrasonic waves allow for the monitoring of critical areas without direct access to thedefect location.During cyclic loading of tensile,aluminum specimens fatigue crack growth at the side of afastener hole was monitored.The changes in the energy ratio of the baseline subtracted reflected guided wavesignal due to the fatigue damage were monitored from a stand-off distance using standard ultrasonic pulse–echo measurement equipment.Good sensitivity for the detection and monitoring of fatigue crack growthwas found.&2015Elsevier Ltd.All rights reserved.1.IntroductionThe development of fatigue cracks at fastener holes in aluminumcomponents is a common maintenance problem for the aircraftindustry[1].Especially the development of widespread fatiguedamage due to stress concentration and cyclic loading conditionsconstitutes a safety-critical problem for ageing aircraft[2].Differentnondestructive testing(NDT)methods have been developed for theearly detection of fatigue cracks[3].Some of them require directaccess to the inside of the fastener hole[3],complicating the possibleintegration into a structural health monitoring(SHM)system.Ultra-sonic bulk wave measurements have a proven track record andsensitivity for the detection and sizing of cracks[4].The real time,in-situ monitoring of fatigue cracks at fastener holes using an anglebeam through transmission technique has been demonstrated[5].However,bulk wave ultrasonic testing necessitates local access to thedamaged area of the inspected structure.Guided ultrasonic waves have been proposed for the integrationinto SHM systems,as with appropriate mode selection they offer therequired area coverage[6].Most work has focused on the selectiveexcitation of one of the fundamental modes(A0and S0Lamb wavemodes)below the cut-off frequencies of the higher wave modes[7],as this allows simpler signal interpretation and typically lowerattenuation for realistic aircraft structures[8].The scattering ofguided waves at a hole with and without a fatigue crack has beeninvestigated[9].Guided waves have been successfully employed todetect and monitor fatigue crack growth in metallic structures[10],and this has been extended for the monitoring of a series of throughholes with multiple crack initiation sites[1].However,low frequencyguided waves have a wavelength significantly larger than in bulkwave ultrasonic testing,ultimately limiting the sensitivity for thedetection of small defects[11].The shorter wavelengths of surfaceacoustic waves propagating along a structure have been employedfor enhanced fatigue crack monitoring sensitivity[12].High frequency guided ultrasonic waves offer an interestingcompromise between the proven defect detection sensitivity of bulkultrasonic waves and achievable propagation range.Different modesand frequency-thickness operating ranges have been investigated fordefect detection over medium long distances, e.g.,for corrosiondetection in aircraft panels[13]and defects in plate structures[14].With the appropriate choice of wave mode excitation good detectionsensitivity for small surface defects and the potential to differentiatethe damaged plate side have been demonstrated[15].Previous workhas found good sensitivity for the early detection and monitoring offatigue crack growth during cyclic loading using noncontact lasermeasurements close to the damage location[16].The two funda-mental Lamb wave modes were excited selectively at a frequency-thickness product of6.75MHz mm,significantly above the cut-offfrequencies of the higher Lamb wave modes[17].The scattering ofthis type of high frequency guided waves from fatigue cracks at aContents lists available at ScienceDirectjournal homepage:/locate/ndteintNDT&E International/10.1016/j.ndteint.2014.12.0070963-8695/&2015Elsevier Ltd.All rightsreserved.n Corresponding author.Tel.:þ442076793944.E-mail address:p.fromme@(P.Fromme).NDT&E International71(2015)1–7fastener hole had been previously measured and compared to numerical predictions in order to understand and quantify the potential detection sensitivity[18].This contribution extends this work to the experimental in-situ monitoring of fatigue crack growth in tensile,aluminum specimens from a stand-off distance from the fastener hole without direct,local access to the damage.The high frequency guided waves were excited using a standard angle beam transducer and monitored using standard ultrasonic pulse–echo equipment.The fatigue crack growth was measured optically during cyclic loading and the changes in the reflected high frequency guided ultrasonic wave pulse were quantified.Calculating the energy ratio of the time-gated and baseline subtracted guided wave reflected pulse–echo(P/E) signal,good sensitivity of the measured changes with crack size were confirmed.2.High frequency guided ultrasonic wave propagationHigh frequency guided waves represent a compromise between achievable propagation distance along plate structures and sensi-tivity for the detection of small defects due to their relative short wavelength[14].For this investigation the fundamental anti-symmetric(A0)and symmetric(S0)Lamb modes at a frequency-thickness region of about6.75MHz mm were excited in a plate (center frequency of2.25MHz for a3mm thick structure).These modes are easily generated and received with sufficient selectivity above the cut-off frequencies of the higher Lamb wave modes using standard angle beam transducers[17].In this frequency region the wavelength equals approximately half of the plate thickness and the A0and S0modes have modeshapes with stress and displacementfields similar to a Rayleigh wave on each plate surface.However,for excitation on the upper surface there is small residual amplitude at the lower boundary,causing a coupling between the two surfaces.This gives rise to an energy transfer from one side of the plate to the other and then back over a distance called the beatlength[19].The beatlength L can be calculated asL¼2πk AÀk S;ð1Þwhere k A0and k Sare the wave numbers of the fundamental anti-symmetric and symmetric Lamb modes.The beatlength depends onthe difference between the wave numbers k A0and k Sin the deno-minator term.With increasing frequency the difference between wave numbers decreases and thus the beatlength increases.For a frequency of2.25MHz mm the difference in wave number(and thus phase velocity)between the fundamental anti-symmetric and symmetric Lamb modes is about0.5%,resulting in a beatlength L¼250mm for a 3mm thick aluminum plate.The associated beating phenomenon can be used for the detection of small cracks on both plate sides with single-sided access or,selecting appropriate excitation frequency and position,for the inspection of structures where access is restricted by regularly spaced features such as stiffeners or stringers[17].Fig.1shows the group velocity dispersion diagram for the used 3mm thick aluminum plate specimens.The group velocities of the fundamental Lamb wave modes(A0and S0)are shown as solid lines. These have been widely employed for SHM applications in large structures at low frequencies[6].In the frequency range of interest around2.25MHz the fundamental modes are rather non-dispersive and the velocities start to converge towards the Rayleigh wave velocity(2918m/s).The group velocity of the A0mode for2.25MHz at2948m/s is slightly higher than the group velocity of the S0mode at2879m/s,resulting in a relative difference of the expected arrival times of about2.5%.3.ExperimentsFive tensile specimens with length600mm,width70mm,and thickness3mm(Fig.2),made of aluminum alloy2014T6,were used for the fatigue testing.A1/4in.diameter hole(r¼3.17mm)was drilled on the center line at200mm from the specimen end(Fig.2). The specimens were subjected to cyclic tensile loading in a servo-hydraulic testing machine(Fig.3(a))with the axis of loading along the specimen length.A maximum load of26kN with stress ratio R¼0.1and a cycling frequency of10Hz were selected.The max-imum stress in the vicinity of the hole(stress concentration factor K t¼2.75)was significantly above the fatigue strength of the alumi-num alloy,but just below the yield limit in order to avoid plastic deformation.A small triangular starter notch,approximately0.2mm long,was made on one side of the specimen at the hole boundary (perpendicular to the loading axis)in order to prescribe the crack initiation location.During fatigue testing the crack grew quarter-elliptically from the starter notch position to a length and depth of about3mm.At that depth,corresponding to the specimen thick-ness,the crack quickly developed into a through-thickness crack.The cyclic loading was paused every1000cycles and the speci-men held under the maximum tensile load to avoid crack closure. The crack size was measured optically on the front surface(crack123456Frequency [MHz]GroupVelocity[m/ms]Fig.1.Group velocity dispersion diagram for3mmthick aluminum plate(Al2014 T6);fundamental modes(A0and S0):solid lines;A1mode:dash-dotted line;higher order modes:dashed lines;center frequency2.25MHz marked.7mm3Fig.2.Schematic of tensile specimen(Al2014T6,600mmÂ70mmÂ3mm)with fastener hole(1/4in./6.35mm diameter)and location of2.25MHz wedge transducer.B.Masserey,P.Fromme/NDT&E International71(2015)1–7 2length)and through the hole thickness (crack depth)by means of an optical microscope and a mirror inserted into the fastener hole.Once the fatigue crack had grown through the thickness,the length on the front and back surface of the specimen were measured and averaged to calculate the crack area (Fig.4(b)).Crack initiation varied between the five specimens,but due to the identical loading conditions the fatigue crack growth was repeatable [16].Typical pictures of the crack growth for one specimen are shown in Fig.3(b)–(d).Fig.3(b)shows the small quarter-elliptical crack at 60,000cycles with a length and depth of approximately 1mm,corresponding to a crack area of 0.88mm 2.For 65,000cycles the quarter-elliptical crack had grown to a depth of about 2mm with a corresponding area of 2.86mm 2(Fig.3(c)).The through thickness crack can be seen in Fig.3(d)at 70,000cycles with a length on the front surface of 3.13mm.The corresponding crack growth data as a function of the number of cycles is shown in Fig.4.The crack was first observed optically at 52,000cycles and had grown through the thickness at 68,000cycles (Fig.4(a)).The experimentally observed fatigue crack growth showed good agreement for crack depth and length with predictions of an analytical model based on Forman crack propaga-tion law [16].Standard ultrasonic pulse –echo (P/E)measurements were con-ducted from a stand-off distance to monitor the high frequency guided wave pulse re flected at the fastener hole and at the fatigue crack.Measurements were taken every 1000cycles while the cyclic loading was paused and the specimen held under maximum load to avoid crack closure during the ultrasonic measurements.Further measure-ments should investigate the relevance of the load (and the resulting crack opening)on the ultrasonic signals and thus detection sensitivity for fatigue cracks.The guided wave pulse was excited and measured using a standard 901angle beam transducer and wedge (Panametrics A540S/ABWML-5T-901)with a center frequency of 2.25MHz (Rayleigh wavelength in aluminum at 2.25MHz:λR ¼1.3mm).As the wedge angle is optimized for steel rather than aluminum and the workingfrequency is signi ficantly above the cut-off frequency of the higher Lamb wave modes (Fig.1)it had been previously found that,besides the desired fundamental Lamb wave modes (A 0and S 0),the first higher antisymmetric Lamb mode (A 1)was excited as well [15].However,the amplitude of the A 1mode was found to be only about 10%of the fundamental modes and due to the dispersion behavior (Fig.1)the wave pulse spreads rapidly in time.In addition,as the group velocity of the A 1mode is about 10%slower than for the A 0mode it arrives signi ficantly after the fundamental modes and can be time-gated out.The wedge was clamped to the tensile specimen (Fig.3(a))at a distance d ¼125mm from the hole center ontheFig.3.(a)Photo of tensile specimen in hydraulic testing machine with microscope and transducer;photos of crack on specimen surface (left)and through hole interior (right):(b)60,000cycles,crack length 1.06mm,crack depth 1.06mm;(c)65,000cycles,crack length 1.81mm,crack depth 2.01mm;(d)70,000cycles,crack length 3.13mm,through thickness.012345C r a c k S i z e [m m]51015Cycles [1’000]C r a c k A r e a [m m 2]Fig.4.Typically observed fatigue crack growth for same specimen as photos in Fig.3;(a)optically measured crack length on specimen surface (dash-dotted)and crack depth in fastener hole interior (solid);(b)calculated crack area;shown against number of cycles.B.Masserey,P.Fromme /NDT &E International 71(2015)1–73specimen surface opposite to the surface where the starter notch wasmade(Fig.2).The distance,corresponding to half a beatlength atcenter frequency(L¼250mm)[16],was selected in order to con-centrate the energy next to the fastener hole on the specimen sidecontaining the starter notch.The transducer was aligned along thespecimen center line to maximize the reflection at the fastener holeand fatigue crack.The specimen width was sufficient so that thesides did not affect the wave propagation,but did not allow for theinvestigation of fatigue cracks at different angles around the fastenerhole relative to the incident wave direction.If the transducer cannotbe placed at an optimum location relative to the expected crackorientation(for the stressfield)the influence on the detectionsensitivity would have to be ascertained.Wave excitation andreception was performed using a standard ultrasonic pulser–receiver(Panametrics5800)to allow for the P/E measurement using theinbuilt receiver circuitry.The excited wave pulse was found to bewideband up to approximately3.5MHz and the internal band-pass filter was set to1–5MHz around the transducer center frequency. The received signals were averaged(100averages)and recordedusing a digital storage oscilloscope(LeCroy9304).4.P/E measurement of fatigue crack growthThe time traces resulting from the pulse–echo measurement at astand-off distance from the fastener hole for the same specimen as inFig.3are shown in Fig.5.The baseline signal in Fig.5(b)shows thereflection at the undamaged fastener hole at the start of the cyclicloading.The zoom of the clear and strong reflected pulse is shown inFig.5(a).The waveform is characterized by a distinct dispersivebehavior.The use of a wideband excitation leads to a partialseparation in time of the A0and S0Lamb wave modes.The arrivaltime of the front of the reflected pulse at about108μs matches the addition of the predicted propagation time in the wedge with thelongitudinal velocity in Perspex and in the specimen with the fastestgroup velocity for the low frequency part of the A0mode,propagat-ing with a group velocity slightly higher than the Rayleigh wavevelocity.The expected arrival time for the S0mode is about2μs later and no time separation of the pulses is visible in Fig.5(a).The main pulse includes both interfering fundamental modes A0and S0.The pulse at about113μs and the following pulses are mainly the low frequency part of the S0mode characterized by a group velocity smaller than the Rayleigh wave velocity.The arrival time of the A1 mode would be about9μs later and as expected no significant pulse at117μs was detected.The time signal shows some amplitude variation between107and120μs,which was consistent between the baseline measurements for the different specimens.Small varia-tions were observed for the initial measurements,possibly due to the settling of the clamped wedge relative to the specimen,and thefirst two recorded time traces at0and1000cycles were discarded.For each specimen,the signal averaged from the next10measurements (2000–11,000cycles)was taken as the baseline signal.Some small changes in the arrival time of the pulses(up to about10%of signal period)were observed before crack initiation,mostly correlated to longer stoppage of the experiments overnight.Therefore,an auto-matic correction to re-align the pulses was implemented in Matlab, using the cross-correlation of the initial reflection of the A0mode at the fastener hole from107to110μs.As the expected arrival time of thefirst reflection at the fatigue crack is about2.5μs later,it was assumed that this part of the pulse was not affected,which was confirmed from visual observation of the reflected pulses.Fig.5(c)shows the reflected time signal recorded for this speci-men at60,000cycles,8000cycles after the fatigue crack wasfirstvisually observed.For a crack length and depth of about1mm achange in the pulse shape with an increase of reflected amplitude inthe later part of the signal can be seen.This change in signal shape and amplitude increased with growing size of the quarter-ellipticalcrack,as shown for a crack depth of about2mm at65,000cycles inFig.5(e).Once the crack had grown through the specimen thicknessa strong reflected pulse was seen as shown in Fig.5(g).It should benoted that the observed changes in the reflected signal varied tosome degree between the different specimens,especially for largercrack areas, e.g.,not for all specimens such a large reflectedamplitude as in Fig.5(g)was seen.As the reflected signals consistof an overlap of wave pulses from different guided wave modes andreflections at the hole and at the fatigue crack,small changes in thelocation and shape of the fatigue crack can lead to relative phaseshifts and thus constructive/destructive interferences of the differentpulses.It was therefore decided to perform a baseline subtraction[18]of the reflected wave pulse relative to the baseline signal(averaged of10measurements).This allowed for the separation ofthe changes in the reflectedfield due to the crack from the directreflection at the hole.The resulting signal is shown in Fig.5(d)forthe1mm long crack at60,000cycles.The time delay of about2.5μs relative to the baseline signal corresponds to the additional propa-gation distance to the fatigue crack location.With increasing cracksize a significant increase in the signal difference can be seen inFig.5(f)for a2mm deep crack at65,000cycles and for a throughthickness crack at70,000cycles in Fig.5(h).5.Energy evaluation of fatigue crack monitoringThe analysis of the measured P/E time traces has shown that therelevant parameter for detection sensitivity is the modification of themeasured waveform due to the additional high frequency guidedwave arising from the reflection at the crack,separated using abaseline subtraction.The time traces shown in Fig.5(c)–(g)shows achange in the pulse shape with an increase of reflected amplitude inthe later part of the signal.Potentially a fatigue crack could have beendetected just from the change in the P/E time signal by itself.However,an energy ratio method similar to[16]was applied to allow a simplequantification of the relative change in the baseline subtracted signaland easier interpretation.This procedure was found to be morereliable than,for example,the maximum pulse amplitude,due tothe interference of the different wave pulses.The energy parameter E nat cycle n was defined as the addition of the energy parameter E0ofthe baseline measurement and the energy parameter E n n of thewaveform at cycle n after baseline subtraction.This parameter wascalculated within a windowed portion of the signal that extendedfrom time t0¼107μs to t1¼120μs containing the complete reflected pulse as explained above.The energy ratio R was obtained by normalizing the energy parameter E n with the baseline parameter E0: R¼E n¼1þEnn;ð2ÞwithE0¼Z t1t0x0ðtÞðÞ2dt;E n n¼Z t1t0x nnðtÞÀÁ2dt;ð3Þwhere t is time,x0(t)is the baseline signal and x nnðtÞis the waveform at cycle n after baseline subtraction.Fig.6(a)shows the calculated energy ratio for the5tensile specimens,with Fig.6(b)giving a magnification of the same data for small crack sizes.The ultrasonic P/E measurement every1000cycles was evaluated as described above and the energy ratio was plotted against the optically measured crack area.The initial crack area corresponds to the length and depth of the starter notch,which varied slightly between specimens.Crack initiation was detected optically at crack lengths varying between0.26mm and0.42mm for the different tensile specimens.Before optical detection,the energy ratio was plotted as if occurring at the area of the starter notch andB.Masserey,P.Fromme/NDT&E International71(2015)1–7 4taken as the uncertainty in the measurements.The largest observed variation in the energy ratio before a fatigue crack was visually detected was 7.3%for one specimen (dash-dotted line),with smaller values from 1.8%to 6.5%for the other 4specimens.The energy ratio increases with increasing crack area,but at slightly different rates for the five specimens.A conservative threshold of 15%increase in the energy ratio was set as twice the maximum variation of 7.3%(Fig.6(b)).For all specimens an increase in energy ratio of more than 15%was observed for a crack area of 0.8mm 2or less.For individual specimens smaller cracks could potentially be detected,but it would not be possible to set speci fic threshold limits in advance.The crack detection threshold of 0.8mm 2corresponds approximately to a crack length and depth of 1mm (approximately 75%of the Rayleigh wavelength in aluminum),similar to the crack at 60,000cycles shown in Fig.3(b).The energy ratio continues to increase with growing crack area.For a crack area of up to 2mm 2reasonably similar behavior of the curves for the five different specimens can be observed in Fig.6(a),even though some differences are evident in the magni fication in Fig.6(b).For small fatigue cracks this might in principle allow an approximate sizing of the crack area from the calculated energy ratio,but with rather large uncertainties.For larger fatigue cracks (42mm 2in Fig.6(a))different rates for the correlation of the energy ratio to the crack can be observed.For 3of the specimens the increase with crack area is larger than for the other 2specimens,making crack sizing impossible.The increased energy ratios matched the observed changes in the time406080100120100110120130100110120130100110120130−0.2−0.100.10.2100110120130100110120130−0.2−0.100.10.2100110120130Time [µs]S i g n a l [V ]100110120130−0.2−0.100.10.2Fig.5.Measured P/E time traces and baseline subtracted signal differences (time window 107–120μs shown);(a)zoom of signal for 10,000cycles,no crack (baseline);(b)signal for 10,000cycles;(c)signal for 60,000cycles,crack length 1.06mm,crack depth 1.06mm;(d)signal difference for 60,000cycles;(e)signal for 65,000cycles,crack length 1.81mm,crack depth 2.01mm;(f)signal difference for 65,000cycles;(g)signal for 70,000cycles,crack length 3.13mm,through thickness;(h)signal difference for 70,000cycles.B.Masserey,P.Fromme /NDT &E International 71(2015)1–75traces, e.g.,signi ficantly larger amplitude changes for some of the specimens compared to the others.No apparent reason for this was observed during the fatigue testing and during the evaluation proce-dure,but the variation between the curves for large fatigue cracks can possibly be associated to small differences in crack location and shape between the measurements.This modi fies the interferences between the A 0and S 0modes re flected at the crack and fastener hole and affects the P/E measurement.The selection of the energy ratio as the detection parameter allows a simple quanti fication of the fatigue crack in fluence on the re flected signal and thus comparison between specimens and setting of a conservative threshold for which small fatigue cracks can be detected from a stand-off distance.6.ConclusionsThe detection and monitoring of fatigue crack growth at fastener holes in aircraft structures presents a signi ficant maintenance problem,where cracks need to be detected before they have reached a safety critical size.Due to their relative short wavelength,high frequency guided waves represent a compromise between achiev-able propagation distance along thin aerospace structures and sensitivity for the detection of small defects.For the integration into SHM systems the sensitivity and reliability of defect detection needs to be ascertained.Previous contributions have demonstrated the effect of a fatigue crack on the scattered wave field and the good sensitivity for the early detection and monitoring of fatigue crack growth employing noncontact measurements using a laser inter-ferometer close to the fastener hole and fatigue crack location.This investigation demonstrated the potential for practical measurements by means of standard ultrasonic pulse –echo equipment at a stand-off distance from the fastener hole without direct access to the damage location.Employing a standard ultrasonic wedge transducer clamped at a stand-off distance from the damage location,the combined re flection at the fastener hole and fatigue crack was measured.The P/E signal contains the superposition of the funda-mental high frequency guided wave modes (A 0and S 0Lamb modes),which overlap in time.The calculation of the energy ratio of the baseline subtracted and time gated signals was employed to quantify the observed changes with fatigue crack ing a conserva-tive limit of twice the maximum observed variation in the energy for any of the five tested specimens,fatigue cracks with an area of less than 0.8mm 2,corresponding approximately to a crack length and depth of 1mm,could be reliably detected.Due to the varying interference between the different re flected guided wave pulsesthe change in the time signals and thus rate of increase in the energy ratio with increasing crack size varied,especially for larger fatigue cracks.This makes a direct correlation of the energy ratio to the crack size dif ficult.Further studies should be performed to investigate this further,as well as to understand the in fluence of load and tempera-ture on the observed signal changes and the applicability of the methodology for complex,multi-layered aircraft structures.AcknowledgementsThis work was partially supported by the UK Engineering and Physical Sciences Research Council (EPSRC)(grant number EP/D065011/1).References[1]Cho H,Lissenden CJ.Structural health monitoring of fatigue crack growth in platestructures with ultrasonic guided waves.Struct Health Monit 2012;11:393–404.[2]Pitt S,Jones R.Multiple-site and widespread fatigue damage in aging aircraft.Eng Fail Anal 1997;4:237–57.[3]Papazian JM,Nardiello J,Silberstein RP,Welsh G,Grundy D,Craven C,et al.Sensors for monitoring early stage fatigue cracking.Int J Fatigue 2007;29:1668–80.[4]Cobb AC,Michaels JE,Michaels TE.An automated time –frequency approachfor ultrasonic monitoring of fastener hole cracks.NDT E Int 2007;40:525–36.[5]Michaels JE,Michaels TE,Mi B.An ultrasonic angle beam method for in situsizing of fastener hole cracks.J Nondestr Eval 2006;25:3–16.[6]Wilcox PD,Lowe MJS,Cawley P.Mode and transducer selection for long rangeLamb wave inspection.J Intell Mater Syst Struct 2001;12:553–65.[7]Fromme P,Wilcox PD,Lowe MJS,Cawley P.On the development and testing ofa guided ultrasonic wave array for structural integrity monitoring.IEEE Trans Ultrason Ferroelectr Freq Control 2006;53:777–85.[8]Dalton RP,Cawley P,Lowe MJS.The potential of guided waves for monitoringlarge areas of metallic aircraft fuselage structures.J Nondestr Eval 2001;20:29–46.[9]Fromme P,Sayir MB.Detection of cracks at rivet holes using guided waves.Ultrasonics 2002;40:199–203.[10]Leong WH,Staszewski WJ,Lee BC,Scarpa F.Structural health monitoring usingscanning laser vibrometry:mb waves for fatigue crack detection.Smart Mater Struct 2005;14:1387–95.[11]Alleyne DN,Cawley P.The interaction of Lamb waves with defects.IEEE TransUltrason Ferroelectr Freq Control 1992;39:381–97.[12]Connolly GD,Rokhlin SI.Enhancement of fatigue crack monitoring by surfaceacoustic wave re flection and modulation in a space-cycle-load domain:an imaging approach.Struct Health Monit 2012;11:187–96.[13]Terrien N,Osmont D,Royer D,Lepoutre F,Déom A.A combined finite elementand modal decomposition method to study the interaction of Lamb modes with micro-defects.Ultrasonics 2007;46:47–78.[14]Greve DW,Zheng P,Oppenheim IJ.The transition from Lamb waves tolongitudinal waves in plates.Smart Mater Struct 2008;17:035029.[15]Masserey B,Fromme P.On the re flection of coupled Rayleigh-like waves atsurface defects in plates.J Acoust Soc Am 2008;123:88–98.123456789E n e r g y R a t i oCrack Area [mm 2]0.20.40.60.8111.051.11.151.21.251.31.35Fig.6.(a)Energy ratio of re flected P/E signal for 5tensile specimens against optically measured crack area (solid lines);time window 107–120μs;(b)zoom for small crack area;7.3%maximum variation before visual crack observation (dash-dotted);15%threshold (dashed).B.Masserey,P.Fromme /NDT &E International 71(2015)1–76。
制作撞蛋的作文英语翻译
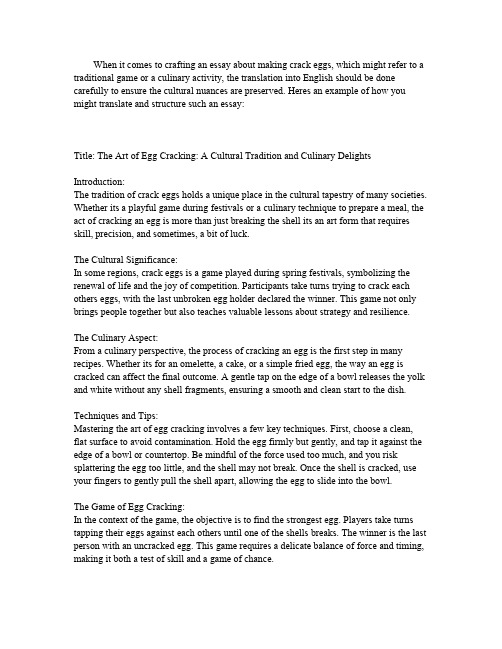
When it comes to crafting an essay about making crack eggs,which might refer to a traditional game or a culinary activity,the translation into English should be done carefully to ensure the cultural nuances are preserved.Heres an example of how you might translate and structure such an essay:Title:The Art of Egg Cracking:A Cultural Tradition and Culinary DelightsIntroduction:The tradition of crack eggs holds a unique place in the cultural tapestry of many societies. Whether its a playful game during festivals or a culinary technique to prepare a meal,the act of cracking an egg is more than just breaking the shell its an art form that requires skill,precision,and sometimes,a bit of luck.The Cultural Significance:In some regions,crack eggs is a game played during spring festivals,symbolizing the renewal of life and the joy of competition.Participants take turns trying to crack each others eggs,with the last unbroken egg holder declared the winner.This game not only brings people together but also teaches valuable lessons about strategy and resilience.The Culinary Aspect:From a culinary perspective,the process of cracking an egg is the first step in many recipes.Whether its for an omelette,a cake,or a simple fried egg,the way an egg is cracked can affect the final outcome.A gentle tap on the edge of a bowl releases the yolk and white without any shell fragments,ensuring a smooth and clean start to the dish. Techniques and Tips:Mastering the art of egg cracking involves a few key techniques.First,choose a clean, flat surface to avoid contamination.Hold the egg firmly but gently,and tap it against the edge of a bowl or countertop.Be mindful of the force used too much,and you risk splattering the egg too little,and the shell may not break.Once the shell is cracked,use your fingers to gently pull the shell apart,allowing the egg to slide into the bowl.The Game of Egg Cracking:In the context of the game,the objective is to find the strongest egg.Players take turns tapping their eggs against each others until one of the shells breaks.The winner is the last person with an uncracked egg.This game requires a delicate balance of force and timing, making it both a test of skill and a game of chance.Conclusion:The act of crack eggs transcends its simple appearance,offering a window into cultural practices and culinary artistry.Whether its a symbol of springs renewal or the foundation of a delicious meal,the humble egg cracking is a tradition that continues to bring people joy and satisfaction.This essay provides a comprehensive look at the concept of crack eggs,covering both its cultural and culinary significance,while also offering practical advice on how to perform the act effectively.。
奥氏体-铁素体型 英文
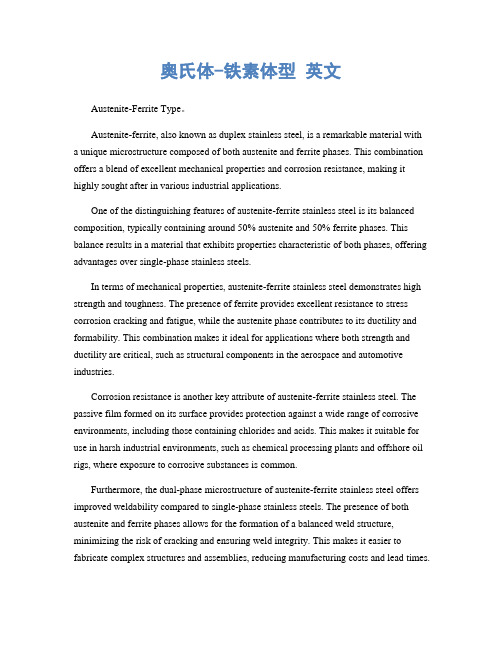
奥氏体-铁素体型英文Austenite-Ferrite Type。
Austenite-ferrite, also known as duplex stainless steel, is a remarkable material with a unique microstructure composed of both austenite and ferrite phases. This combination offers a blend of excellent mechanical properties and corrosion resistance, making it highly sought after in various industrial applications.One of the distinguishing features of austenite-ferrite stainless steel is its balanced composition, typically containing around 50% austenite and 50% ferrite phases. This balance results in a material that exhibits properties characteristic of both phases, offering advantages over single-phase stainless steels.In terms of mechanical properties, austenite-ferrite stainless steel demonstrates high strength and toughness. The presence of ferrite provides excellent resistance to stress corrosion cracking and fatigue, while the austenite phase contributes to its ductility and formability. This combination makes it ideal for applications where both strength and ductility are critical, such as structural components in the aerospace and automotive industries.Corrosion resistance is another key attribute of austenite-ferrite stainless steel. The passive film formed on its surface provides protection against a wide range of corrosive environments, including those containing chlorides and acids. This makes it suitable for use in harsh industrial environments, such as chemical processing plants and offshore oil rigs, where exposure to corrosive substances is common.Furthermore, the dual-phase microstructure of austenite-ferrite stainless steel offers improved weldability compared to single-phase stainless steels. The presence of both austenite and ferrite phases allows for the formation of a balanced weld structure, minimizing the risk of cracking and ensuring weld integrity. This makes it easier to fabricate complex structures and assemblies, reducing manufacturing costs and lead times.In addition to its mechanical and corrosion-resistant properties, austenite-ferrite stainless steel exhibits good thermal stability and resistance to thermal expansion. This makes it suitable for use in high-temperature applications, such as heat exchangers and furnace components, where dimensional stability is crucial.Despite its many advantages, austenite-ferrite stainless steel also has some limitations. One challenge is maintaining the balance between the austenite and ferrite phases during fabrication and heat treatment processes. Any deviation from the desired phase balance can affect the material's properties, leading to reduced performance and reliability.In conclusion, austenite-ferrite stainless steel offers a unique combination of mechanical strength, corrosion resistance, and weldability, making it a versatile material for various industrial applications. Its dual-phase microstructure provides a balance of properties that are not achievable with single-phase stainless steels, making it an attractive choice for engineers and designers looking to optimize performance and reliability in their projects.。
基于振动疲劳的某水泵支架开裂分析及结构优化
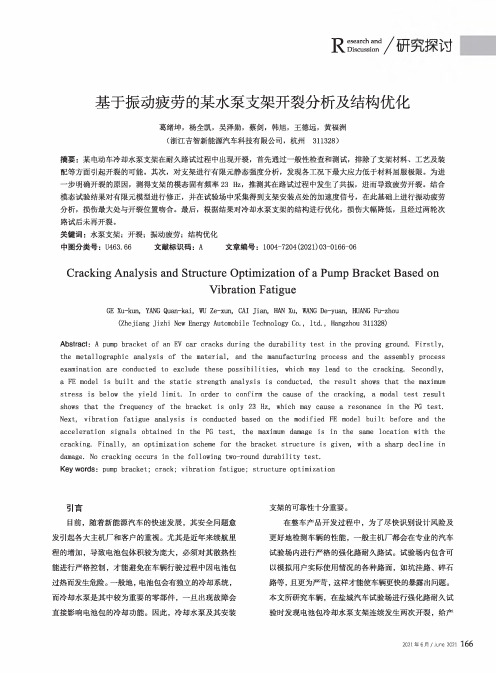
分析,损伤最大处与开裂位置吻合。最后,根据结果对冷却水泵支架的结构进行优化,损伤大幅降低,且经过两轮次
路试后未再开裂。
关键词:水泵支架;开裂;振动疲劳;结构优化
中图分类号:U463. 66
文献标识码:A
文章编号:1004-7204(2021)03-0166-06
Cracking Analysis and Structure Optimization of a Pump Bracket Based on Vibration Fatigue
冷却水泵
安装支架
P1 图3冷却水泵支架结构示意图
167 环境技术 / Environmental Technology
Research and / Discussion / W\
kU
架,可以发现:冷却水泵质心偏离两个螺栓安装点中心 线较多,安装点P2距离质心更近,所以承担载荷更大, 但结构却比较薄弱,很有可能是该原因导致的开裂。
2021 年 6月 /June 2021 166
Vt / TP esearch and UTT九怀 ku / lx Discussion
图1冷却水泵支架两次开裂位置
表1样件检测结果
序号
试验项目
试验标准
1
化学成分
GB/T 4336-2002
2
金相分析
GB/T 13298-2015
厂 材料成分问题——金相组织不合理
Research and / Discussion /
\+ HI
基于振动疲劳的某水泵支架开裂分析及结构优化
葛绪坤,杨全凯,吴泽勋,蔡剑,韩旭,王德远,黄福洲 (浙江吉智新能源汽车科技有限公司,杭州311328)
摘要:某电动车冷却水泵支架在耐久路试过程中出现开裂,首先通过一般性检查和测试,排除了支架材料、工艺及装
U71Mn钢轨闪光焊对接接头断裂原因分析
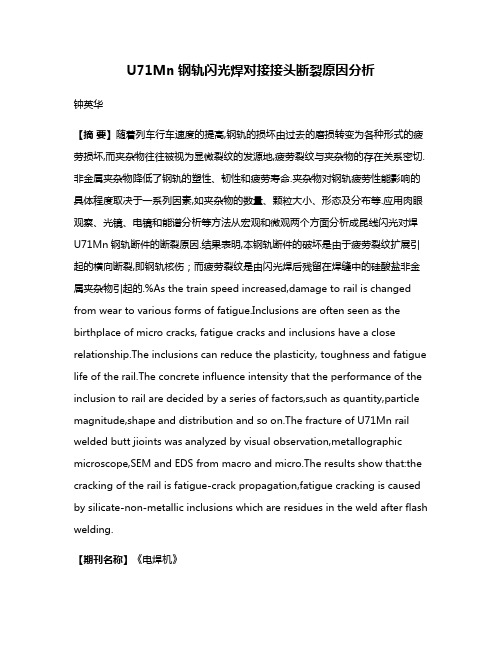
U71Mn钢轨闪光焊对接接头断裂原因分析钟英华【摘要】随着列车行车速度的提高,钢轨的损坏由过去的磨损转变为各种形式的疲劳损坏,而夹杂物往往被视为显微裂纹的发源地,疲劳裂纹与夹杂物的存在关系密切.非金属夹杂物降低了钢轨的塑性、韧性和疲劳寿命.夹杂物对钢轨疲劳性能影响的具体程度取决于一系列因素,如夹杂物的数量、颗粒大小、形态及分布等.应用肉眼观察、光镜、电镜和能谱分析等方法从宏观和微观两个方面分析成昆线闪光对焊U71Mn钢轨断件的断裂原因.结果表明,本钢轨断件的破坏是由于疲劳裂纹扩展引起的横向断裂,即钢轨核伤;而疲劳裂纹是由闪光焊后残留在焊缝中的硅酸盐非金属夹杂物引起的.%As the train speed increased,damage to rail is changed from wear to various forms of fatigue.Inclusions are often seen as the birthplace of micro cracks, fatigue cracks and inclusions have a close relationship.The inclusions can reduce the plasticity, toughness and fatigue life of the rail.The concrete influence intensity that the performance of the inclusion to rail are decided by a series of factors,such as quantity,particle magnitude,shape and distribution and so on.The fracture of U71Mn rail welded butt jioints was analyzed by visual observation,metallographic microscope,SEM and EDS from macro and micro.The results show that:the cracking of the rail is fatigue-crack propagation,fatigue cracking is caused by silicate-non-metallic inclusions which are residues in the weld after flash welding.【期刊名称】《电焊机》【年(卷),期】2011(041)012【总页数】5页(P48-52)【关键词】U71Mn钢轨;疲劳断裂;硅酸盐夹杂【作者】钟英华【作者单位】成都铁路局工务处,四川成都 610082【正文语种】中文【中图分类】TG441.7近年来,我国高速铁路快速发展,特别是客专行车速度不断提高。