A comparison of bulk cable current injection and radiated field susceptibility test methods
酸碱浓度变送器AX43说明书
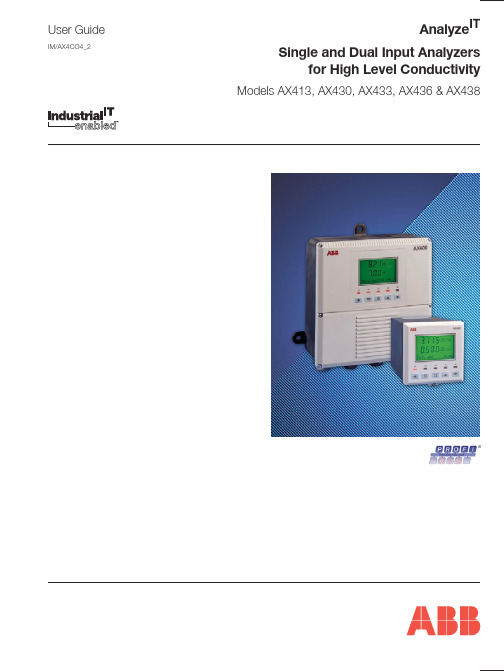
Health and Safety To ensure that our products are safe and without risk to health, the following points must be noted: 1. The relevant sections of these instructions must be read carefully before proceeding. 2. Warning labels on containers and packages must be observed. 3. Installation, operation, maintenance and servicing must only be carried out by suitably trained personnel and in accordance with the information given. 4. Normal safety precautions must be taken to avoid the possibility of an accident occurring when operating in conditions of high pressure and/ or temperature. 5. Chemicals must be stored away from heat, protected from temperature extremes and powders kept dry. Normal safe handling procedures must be used. 6. When disposing of chemicals ensure that no two chemicals are mixed. Safety advice concerning the use of the equipment described in this manual or any relevant hazard data sheets (where applicable) may be obtained from the Company address on the back cover, together with servicing and spares information.
低压电工各地区题目

低压电工各地区题目英文回答:1. General Electrical Knowledge.What are the different types of electrical circuits? What are the basic principles of electricity?How do you calculate the resistance of a circuit?How do you calculate the current flow in a circuit?What are the different types of electrical components?2. Electrical Safety.What are the general safety rules for working with electricity?What are the specific hazards of working with low-voltage electricity?What are the proper procedures for testing electrical circuits?What are the proper procedures for installing electrical equipment?What are the proper procedures for troubleshooting electrical problems?3. Electrical Wiring.What are the different types of electrical wiring?How do you select the correct type of wire for a particular application?How do you properly install electrical wiring?How do you terminate electrical wires?How do you troubleshoot electrical wiring problems?4. Electrical Equipment.What are the different types of electrical equipment?How do you select the correct type of equipment for a particular application?How do you properly install electrical equipment?How do you maintain electrical equipment?How do you troubleshoot electrical equipment problems?5. Electrical Codes and Standards.What are the different electrical codes and standards? How do you comply with electrical codes and standards?What are the consequences of not complying with electrical codes and standards?中文回答:1. 电工基础知识。
高三英语通讯技术词汇综合单选题40题
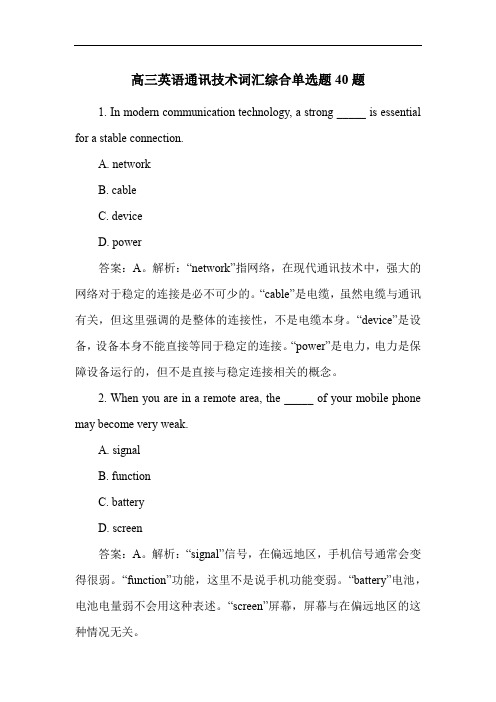
高三英语通讯技术词汇综合单选题40题1. In modern communication technology, a strong _____ is essential for a stable connection.A. networkB. cableC. deviceD. power答案:A。
解析:“network”指网络,在现代通讯技术中,强大的网络对于稳定的连接是必不可少的。
“cable”是电缆,虽然电缆与通讯有关,但这里强调的是整体的连接性,不是电缆本身。
“device”是设备,设备本身不能直接等同于稳定的连接。
“power”是电力,电力是保障设备运行的,但不是直接与稳定连接相关的概念。
2. When you are in a remote area, the _____ of your mobile phone may become very weak.A. signalB. functionC. batteryD. screen答案:A。
解析:“signal”信号,在偏远地区,手机信号通常会变得很弱。
“function”功能,这里不是说手机功能变弱。
“battery”电池,电池电量弱不会用这种表述。
“screen”屏幕,屏幕与在偏远地区的这种情况无关。
3. The development of communication technology has made the global _____ much closer.A. linkB. wireC. chipD. keyboard答案:A。
解析:“link”有连接、联系的意思,通讯技术的发展让全球联系更加紧密。
“wire”电线,侧重于物理的电线,不能体现全球联系。
“chip”芯片,芯片只是通讯技术中的一部分,不能代表整体的全球联系。
“keyboard”键盘,与全球联系没有关系。
4. In a wireless communication system, the _____ between devices needs to be well - coordinated.A. communicationB. interferenceC. distanceD. software答案:A。
理工英文考试题及答案解析
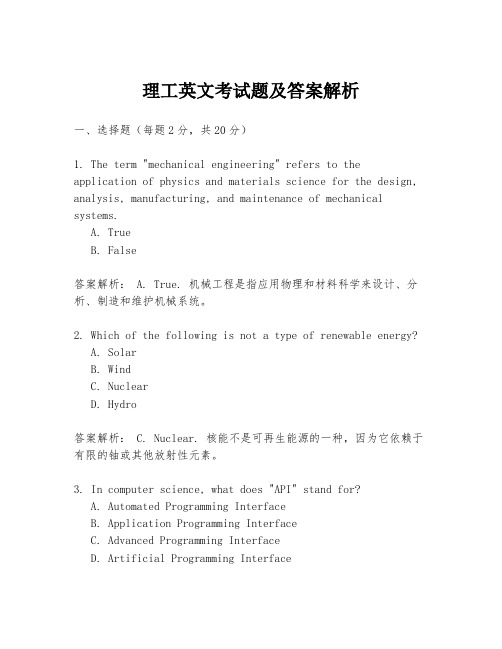
理工英文考试题及答案解析一、选择题(每题2分,共20分)1. The term "mechanical engineering" refers to the application of physics and materials science for the design, analysis, manufacturing, and maintenance of mechanical systems.A. TrueB. False答案解析: A. True. 机械工程是指应用物理和材料科学来设计、分析、制造和维护机械系统。
2. Which of the following is not a type of renewable energy?A. SolarB. WindC. NuclearD. Hydro答案解析: C. Nuclear. 核能不是可再生能源的一种,因为它依赖于有限的铀或其他放射性元素。
3. In computer science, what does "API" stand for?A. Automated Programming InterfaceB. Application Programming InterfaceC. Advanced Programming InterfaceD. Artificial Programming Interface答案解析: B. Application Programming Interface. API 是应用程序编程接口的缩写。
4. The formula for calculating the area of a circle is A = πr², where "r" represents:A. The diameter of the circleB. The circumference of the circleC. The radius of the circleD. The area of the circle答案解析: C. The radius of the circle. 圆的面积公式 A =πr²中的 "r" 表示圆的半径。
三星品牌钽电容说明书

�
Domestic Distributors
KORCHIP INC #219 - 8 Gasan - dong, Gumchun - gu, Seoul, Korea Te l: +82 - 2 - 838 - 5588 E-mail:hjh0064@ CHUNG HAN #16-96 Hangang - lo 3, Youngsan - Gu, Seoul, Korea Te l: +82 - 2 - 718 - 3322 E-mail:james - kim@ichunghan.co.kr SAMTEK #154-16 Samsung- dong Kangnam- Gu, Seoul, Korea Te l: +82- 2- 3458- 9340 E-mail:sinsog1@samtek.co.kr CHUNGMAC #53-5 Wonhyolo3 Youngsan- gu, Seoul, Korea Te l: +82- 2- 716 - 6428~9 E-mail:any@anycam.co.kr APEXINT #1258 Gulo - dong Gulo-Gu, Seoul, Korea Te l: +82- 2- 6679- 5116, 5118 E-mail:djlee@apexint.co.kr
�
Manufacturing Site
Suwon Plant 314, Maetan 3- dong, Youngtong- gu, Suwon, Kyonggi Province 442- 743, Korea Te l: +82- 31- 218- 2063 E-mail:sh386.kim@ Pusan Plant 1623-2, Songjong-dong, Kangso - gu, Pusan 618 - 270, Korea Te l: +82- 51- 970 - 7741 E-mail:absyong.kim@ Tianjin Plant 27, Heiniu, Cheng - Road, Tianjin, China 300210 Te l: +86 - 22 - 2830 - 3333(3450) E-mail:gk.ryu@ Philippines Plant Calamba Premiere International Park, Batino, Calamba, Laguna, manila Te l: +63 - 2 - 809 - 2873 E-mail:ksj1445@
顾问必须熟悉的ERP专业术语中英及缩写对照
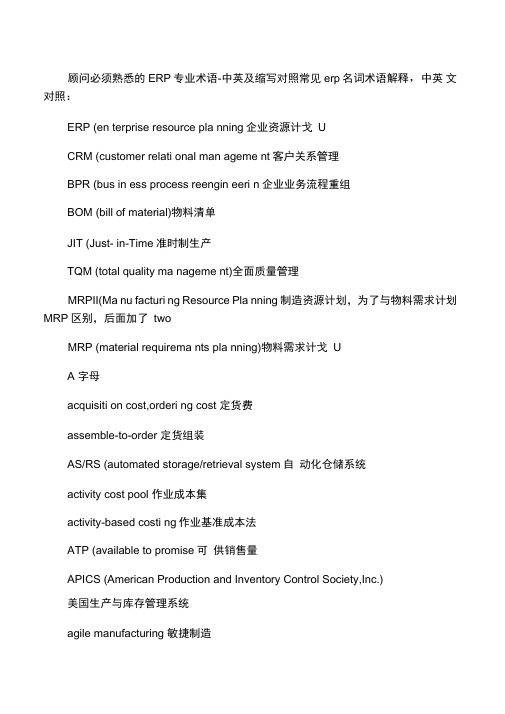
顾问必须熟悉的ERP专业术语-中英及缩写对照常见erp名词术语解释,中英文对照:ERP (en terprise resource pla nning企业资源计戈UCRM (customer relati onal man ageme nt客户关系管理BPR (bus in ess process reengin eeri n企业业务流程重组BOM (bill of material)物料清单JIT (Just- in-Time准时制生产TQM (total quality ma nageme nt)全面质量管理MRPII(Ma nu facturi ng Resource Pla nning制造资源计划,为了与物料需求计划MRP区别,后面加了twoMRP (material requirema nts pla nning)物料需求计戈UA 字母acquisiti on cost,orderi ng cost 定货费assemble-to-order 定货组装AS/RS (automated storage/retrieval system自动化仓储系统activity cost pool 作业成本集activity-based costi ng作业基准成本法ATP (available to promise可供销售量APICS (American Production and Inventory Control Society,Inc.)美国生产与库存管理系统agile manufacturing 敏捷制造APICS Applied Manu facturi ng Education Serie实用制造管理系列培训教材AMT (Adva need Manu factur ing Tech nology先进制造技术anticipation inventory 预期储备B 字母back scheduli ng 倒排计划backflushing 反冲法BOR (bill of resource 资源清单bus in ess pla n 经营规戈U batch process 批流程bottleneck 瓶颈资源(工序) back order 脱期定单backlog 拖欠定单bill of materials 物料清单bucketless syste m 无时段系统C 字母carry ing cost 保管费closed-loop MRP闭环MRP cost roll-up 成本滚动计算法costed BOM成本物料单cost of stockout 短缺损失critical work center 关键工作中心critical path method 关键路线法CAPP (computer-aided process pla nning计算机辅助工艺设计CASE (computer-aided software engin eeri ng计算机辅助软件工程CAD (computer-aided design)计算机辅助设计CAM (computer-aided man ufacturi ng)计算机辅助制造CIMS (computer integrated manufacturing system)计算机集成制造系统critical ratio 紧迫系数component 子件/组件cost driver rate 作业成本发生因素单位费用cost driver 作业成本发生因素customer deliver leadtime 客户交货提前期cumulative lead time 累计提前期con ti nu ous process 连续流程COMMS (Customer Orie nted Manu facturi ng Ma nageme nt System面向客户制造管理系统capacity level 能力利用水平capacity man ageme nt 能力管理capacity requirements planning 能力需求计划current standart cost 现行标准成本cycle counting 循环盘点D 字母DCS (distributed control system分布式控制系统DMRP (distributed MRP分布式MRPDRP (distributio n resource pla nnin g分销资源计划distribution requirementsplanning 分销需求计划demonstrated capacity 纪实能力decision supportsystem 决策支持系统discrete manufacturing 离散型生产dispatch list 派工单days offset 偏置天数dependent demand 相关需求件dema nd man ageme nt 需求管理DTF (demand time f en ce)需求时界dema nd cycle需求周期dropshipment 直运E字母EDI (electro nic data in tercha nge电子数据交换ergonomics 工效学EOQ (ec ono mic order qua ntity经济定货量法earliest due date 最早定单完成日期ECO (engin eeri ng cha nge order/ notice 设计变更通知engineering BOM 工程物料清单ETO (en gi neer-to-order专项生产F字母financial accounting 财务会计financial entity 财务实体fixed period requirements 定期用量法FOQ (fixed order qua ntity)固定批量法formal system 规范化管理系统feature 基本组件floor stock,bulk item 作业现场库存firm-planned order 确定定单firm-planned time fence 确定计划时单FMS (flexible man ufacturi ng system柔性制造系统feature 特征件forward scheduling 顺排计划favorable varia nee 有利差异FCS (finite capacity scheduli ng有限能力计划finite forward scheduling 有限顺排计划finite loading 有限排负荷FAS (final assembly schedule总、装进度G字母GT (group tech no logy成组技术gross requirements 毛需求H 字母hedge inventory 囤积库存I 字母independent demand 独立需求informal system 非规范化管理inventory 库存inventory turnover/turns 库存(资金)周转次数indented BOM 缩排式物料清单input/output control 投入/产出控制item,material,part 物料item master,material master 物料主文件infinite loading 无限排负荷J字母job shop 机群式布置车间K字母kitting 配套出售件L字母low-lever code 低层码logistics 后勤保证体系lean production 精益生产least slack per operation 最小单个工序平均时差lot sizing 批量规则lot size inventory 批量库存lead time 提前期lead time offset 提前期偏置lot-for-lot 因需定量法live pilot 应用模拟M 字母move time 传送时间MTO (make-to-order)定货生产management accounting 管理会计MIS (ma nageme nt in formatio n system)管理信息系统minimum balance 最小库存余量management by exception 例外管理法modular BOM 模块化物料单measure of velocity生产速率水平maintenance,repair,and operation supplies 维护修理操作物料material management 物料管理material review board 物料核定机构material manager 物料经理material available 物料可用量Modern Materials Handling 现代物料搬运manufacturing BOM 制造物料清单MES (ma nu facturi ng executive system 制造执行系统MPS (master production schedule 庄生产计划master scheduler主生产计戈U员N 字母net cha nge净改变法net requirements 净需求netting 净需求计算O 字母order policy 定货策略order point system 定货点法ordering cost 定货费overhead apportionment/allocation 间接费分配overhead rate,burden factor,absorption rate 间按费率option 可选件open order 未结定单OPTO (ptimized Productio n Tech no logy)优化生产技术P 字母ploicy and procedure 工作准则与工作规程planned order receipts 计划产出量planned order 计划定单planned capacity 计划能力PERT (program evaluati on research tech no logy计戈U评审技术planning horizon 计划期PTF (pla nned time fen ce 计划时界pla nned order releases 计划投入量planning BOM 计划物料单proposed cost 建议成本pick ing list领料/提货单parent item 母件Pareto Prin ciple帕拉图原理production cycle 生产周期production activity control 生产作业控制point of use 使用点phantom 虚拟件performance measurement 业绩评价projected available bala nee 预计可用库存量priority 优先级prototyping,computer pilot 原型测试POQ (period order quan tity)周期定货量法pegging 追溯Q 字母queue time 排队时间R字母resupply order 补库单RCCP (rough-cut capacity pla nninj粗能力计划repetitive manufacturing 重复式生产rated capacity 额定能力routing 工艺路线run time 加工时间resource requirements planning 资源需求计划requisition 请购单regeneration 全重排法released order ,open order 下达定单required capacity 需用能力S字母safety stock 安全库存safety lead time 安全提前期sta ndard cost system标准成本体系shop floor control 车间作业控制shop order 车间定单scrap 废品率scheduler 计划员supply chain 供需链shop calendar 工作日历summarized BOM 汇总物料清单scheduled receipts 计划接收量seas onal stock 季节储备ship-to 交货地set up time 准备时间spe nding varia nce,expe nditure varia nee 开支差异SMED (si ngle-mi nute excha nge of dies快速换模法simulated cost 模拟成本shri nkage缩减率synchronous manufacturing 同步制造SOP (sales and operati ons pla nning销售与动作规划T 字母transit time 传送时间top management commitment 领导承诺time bucket 时段time fence 时界time zone 时区TOC (Theory of Con strai nts约束理论transportation inventory,pipeline stock 在途库存total lead time 总提前期U 字母V 字母volume variance 产量差异value chain 价值链virtual organization 虚拟企业value-added cha in 增值链W 字母work order 车间定单wait time 等待时间work flow 工作流work center 工作中心world class manu facturi ng excelle nee 国际优秀制造业what-if 如果怎样-将会怎样X 字母丫字母yield 成品率。
EX250系列CANopen字段总线设备操作手册说明书
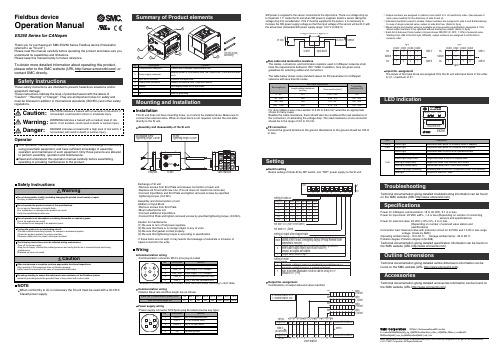
Fieldbus deviceOperation ManualEX250 Series for CANopenMounting and InstallationInstallationThe SI unit does not have mounting holes, so it cannot be installed alone. Make sure to connect the solenoid valve. When an input block is not required, connect the end plate directly to the SI unit.SettingExchange of SI unit•Remove screws from End Plate and release connection of each unit.•Replace old SI unit with new one. (Tie rod does not need to be removed.)•Connect Input Block and End Plate and tighten removed screws by specified tightening torque. (0.6 Nm)Assembly and disconnection of unit Addition of Input Block•Remove screws from End Plate.•Mount attached tie rod.•Connect additional Input Block.•Connect End Plate and tighten removed screws by specified tightening torque. (0.6 Nm)Caution for maintenance(1) Be sure to turn-off all power supplies.(2) Be sure that there is no foreign object in any of units.(3) Be sure that gasket is lined properly.(4) Be sure that tightening torque is according to specification.If these items are not kept, it may lead to the breakage of substrate or intrusion of liquid or dust into the units.TroubleshootingTechnical documentation giving detailed troubleshooting information can be found on the SMC website (URL ).SpecificationsPower for CANopen communication: 18 to 30 VDC, 0.1 A or lessPower for input block: 24 VDC ±20%, 1 A or less (Depending on number of connectingsensors and specifications)Power for solenoid valve: 24 VDC +10%/-5%, 2 A or less(Depending on number of solenoid valve station and specifications)Connection load: Solenoid valve with protection circuit for 24 VDC and 1.5 W or less surgevoltage. (made by SMC)Operating ambient temp: -10 to 50 C Storage ambient temp: -20 to 60 C Pollution degree: Pollution degree 3 (UL508)Technical documentation giving detailed specification information can be found on the SMC website (URL ).Outline DimensionsTechnical documentation giving detailed outline dimensions information can be found on the SMC website (URL ).Assembly and disassembly of the SI unitNOTEWhen conformity to UL is necessary the SI unit must be used with a UL1310Class2 power supply.Thank you for purchasing an SMC EX250 Series Fieldbus device (Hereinafter referred to as "SI unit" ).Please read this manual carefully before operating the product and make sure you understand its capabilities and limitations.Please keep this manual handy for future reference.To obtain more detailed information about operating this product,please refer to the SMC website (URL ) or contact SMC directly.These safety instructions are intended to prevent hazardous situations and/or equipment damage.These instructions indicate the level of potential hazard with the labels of"Caution", " Warning" or "Danger". They are all important notes for safety and must be followed in addition to International standards (ISO/IEC) and other safety regulations.OperatorWiringCommunication wiringSW power is supplied to the sensor connected to the input block. There is a voltage drop up to maximum 1 V inside the SI unit when SW power is supplied. Select a sensor taking this voltage drop into consideration. If 24 V must be supplied to the sensor, it is necessary toincrease the SW power supply voltage so that the input voltage of the sensor will be 24 V with the actual load. (Allowable SW power supply range: 19.2 V to 28.8 V)Bus cable and termination resistorsThe cables, connectors, and termination resistors used in CANopen networks shall meet the requirements defined in ISO 11898. In addition, here are given some guidelines for selecting cables and connectors.The table below shows some standard values for DC parameters for CANopen networks with less than 64 nodes.Switch settingBefore setting of Node-ID by DIP switch, turn “OFF” power supply to the SI unit.Output No. assignmentCombinations of output data and valve manifoldNote: Specifications are subject to change without prior notice and any obligation on the part of the manufacturer.© 2011 SMC Corporation All Rights ReservedtkihabaraMUwXMdhy]Mg_dg_d]MSotokanda]Mvhiyoda_ku]MTokyoMdcd_cced]M±tPt−PhonemM[kdMf_hecj_keglMMMyaxmM[kdMf_helk_hfieUR“Mhttpmbbwwwasmcworldacom∗: Output numbers are assigned to stations from side D to U of manifold in order. (See manual of each valve manifold for the directions of side D and U).∗: Standard manifold is wired in double. Output numbers are assigned to side A and B alternatively.In case of single solenoid valve, output on side B is free. (Refer to fig.a)∗: Mixed (single and double) wiring is available as long as wiring specifications designate it. This allows output numbers to be specified without having free output. (Refer to fig.b)∗: Each bit of data sent from master (4 bytes) shows ON/OFF (0: OFF, 1: ON) of solenoid valve.Starting from LSB of the first byte (Offset0), output numbers are assigned to all the bits in numeric order.Input No. assignmentThe inputs of the Input block are assigned from the SI unit side Input block in the order 0,1,2…maximum of 31.AccessoriesTechnical documentation giving detailed accessories information can be found on the SMC website (URL ).Communication wiringRelation Baud rate and Bus length are as follows.Power supply wiringLED indicationFE connectionConnect the ground terminal to the ground. Resistance to the ground should be 100 Ωor less.For drop cables a wire cross-section of 0.25 to 0.34 mm would be an appropriate choice in many cases.Besides the cable resistance, there should also be considered the real resistance of the connectors, if calculating the voltage drop. The read resistance of one connector should be in the range of 2.5 to 10 mΩ.。
热红外传感史
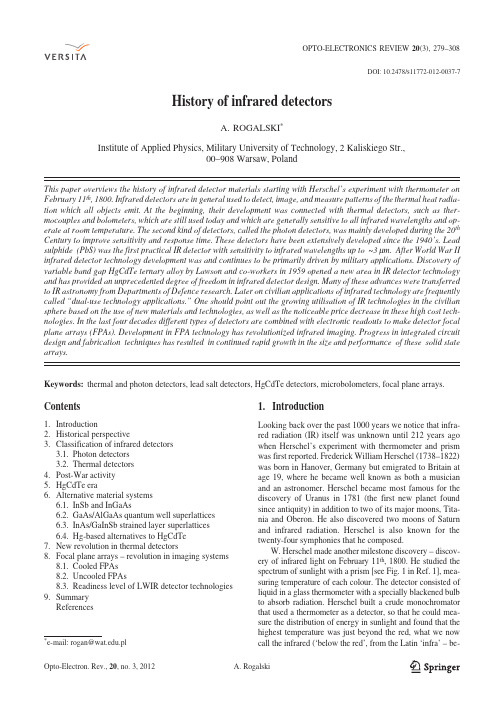
History of infrared detectorsA.ROGALSKI*Institute of Applied Physics, Military University of Technology, 2 Kaliskiego Str.,00–908 Warsaw, PolandThis paper overviews the history of infrared detector materials starting with Herschel’s experiment with thermometer on February11th,1800.Infrared detectors are in general used to detect,image,and measure patterns of the thermal heat radia−tion which all objects emit.At the beginning,their development was connected with thermal detectors,such as ther−mocouples and bolometers,which are still used today and which are generally sensitive to all infrared wavelengths and op−erate at room temperature.The second kind of detectors,called the photon detectors,was mainly developed during the20th Century to improve sensitivity and response time.These detectors have been extensively developed since the1940’s.Lead sulphide(PbS)was the first practical IR detector with sensitivity to infrared wavelengths up to~3μm.After World War II infrared detector technology development was and continues to be primarily driven by military applications.Discovery of variable band gap HgCdTe ternary alloy by Lawson and co−workers in1959opened a new area in IR detector technology and has provided an unprecedented degree of freedom in infrared detector design.Many of these advances were transferred to IR astronomy from Departments of Defence ter on civilian applications of infrared technology are frequently called“dual−use technology applications.”One should point out the growing utilisation of IR technologies in the civilian sphere based on the use of new materials and technologies,as well as the noticeable price decrease in these high cost tech−nologies.In the last four decades different types of detectors are combined with electronic readouts to make detector focal plane arrays(FPAs).Development in FPA technology has revolutionized infrared imaging.Progress in integrated circuit design and fabrication techniques has resulted in continued rapid growth in the size and performance of these solid state arrays.Keywords:thermal and photon detectors, lead salt detectors, HgCdTe detectors, microbolometers, focal plane arrays.Contents1.Introduction2.Historical perspective3.Classification of infrared detectors3.1.Photon detectors3.2.Thermal detectors4.Post−War activity5.HgCdTe era6.Alternative material systems6.1.InSb and InGaAs6.2.GaAs/AlGaAs quantum well superlattices6.3.InAs/GaInSb strained layer superlattices6.4.Hg−based alternatives to HgCdTe7.New revolution in thermal detectors8.Focal plane arrays – revolution in imaging systems8.1.Cooled FPAs8.2.Uncooled FPAs8.3.Readiness level of LWIR detector technologies9.SummaryReferences 1.IntroductionLooking back over the past1000years we notice that infra−red radiation(IR)itself was unknown until212years ago when Herschel’s experiment with thermometer and prism was first reported.Frederick William Herschel(1738–1822) was born in Hanover,Germany but emigrated to Britain at age19,where he became well known as both a musician and an astronomer.Herschel became most famous for the discovery of Uranus in1781(the first new planet found since antiquity)in addition to two of its major moons,Tita−nia and Oberon.He also discovered two moons of Saturn and infrared radiation.Herschel is also known for the twenty−four symphonies that he composed.W.Herschel made another milestone discovery–discov−ery of infrared light on February11th,1800.He studied the spectrum of sunlight with a prism[see Fig.1in Ref.1],mea−suring temperature of each colour.The detector consisted of liquid in a glass thermometer with a specially blackened bulb to absorb radiation.Herschel built a crude monochromator that used a thermometer as a detector,so that he could mea−sure the distribution of energy in sunlight and found that the highest temperature was just beyond the red,what we now call the infrared(‘below the red’,from the Latin‘infra’–be−OPTO−ELECTRONICS REVIEW20(3),279–308DOI: 10.2478/s11772−012−0037−7*e−mail: rogan@.pllow)–see Fig.1(b)[2].In April 1800he reported it to the Royal Society as dark heat (Ref.1,pp.288–290):Here the thermometer No.1rose 7degrees,in 10minu−tes,by an exposure to the full red coloured rays.I drew back the stand,till the centre of the ball of No.1was just at the vanishing of the red colour,so that half its ball was within,and half without,the visible rays of theAnd here the thermometerin 16minutes,degrees,when its centre was inch out of the raysof the sun.as had a rising of 9de−grees,and here the difference is almost too trifling to suppose,that latter situation of the thermometer was much beyond the maximum of the heating power;while,at the same time,the experiment sufficiently indi−cates,that the place inquired after need not be looked for at a greater distance.Making further experiments on what Herschel called the ‘calorific rays’that existed beyond the red part of the spec−trum,he found that they were reflected,refracted,absorbed and transmitted just like visible light [1,3,4].The early history of IR was reviewed about 50years ago in three well−known monographs [5–7].Many historical information can be also found in four papers published by Barr [3,4,8,9]and in more recently published monograph [10].Table 1summarises the historical development of infrared physics and technology [11,12].2.Historical perspectiveFor thirty years following Herschel’s discovery,very little progress was made beyond establishing that the infrared ra−diation obeyed the simplest laws of optics.Slow progress inthe study of infrared was caused by the lack of sensitive and accurate detectors –the experimenters were handicapped by the ordinary thermometer.However,towards the second de−cade of the 19th century,Thomas Johann Seebeck began to examine the junction behaviour of electrically conductive materials.In 1821he discovered that a small electric current will flow in a closed circuit of two dissimilar metallic con−ductors,when their junctions are kept at different tempera−tures [13].During that time,most physicists thought that ra−diant heat and light were different phenomena,and the dis−covery of Seebeck indirectly contributed to a revival of the debate on the nature of heat.Due to small output vol−tage of Seebeck’s junctions,some μV/K,the measurement of very small temperature differences were prevented.In 1829L.Nobili made the first thermocouple and improved electrical thermometer based on the thermoelectric effect discovered by Seebeck in 1826.Four years later,M.Melloni introduced the idea of connecting several bismuth−copper thermocouples in series,generating a higher and,therefore,measurable output voltage.It was at least 40times more sensitive than the best thermometer available and could de−tect the heat from a person at a distance of 30ft [8].The out−put voltage of such a thermopile structure linearly increases with the number of connected thermocouples.An example of thermopile’s prototype invented by Nobili is shown in Fig.2(a).It consists of twelve large bismuth and antimony elements.The elements were placed upright in a brass ring secured to an adjustable support,and were screened by a wooden disk with a 15−mm central aperture.Incomplete version of the Nobili−Melloni thermopile originally fitted with the brass cone−shaped tubes to collect ra−diant heat is shown in Fig.2(b).This instrument was much more sensi−tive than the thermometers previously used and became the most widely used detector of IR radiation for the next half century.The third member of the trio,Langley’s bolometer appea−red in 1880[7].Samuel Pierpont Langley (1834–1906)used two thin ribbons of platinum foil connected so as to form two arms of a Wheatstone bridge (see Fig.3)[15].This instrument enabled him to study solar irradiance far into its infrared region and to measure theintensityof solar radia−tion at various wavelengths [9,16,17].The bolometer’s sen−History of infrared detectorsFig.1.Herschel’s first experiment:A,B –the small stand,1,2,3–the thermometers upon it,C,D –the prism at the window,E –the spec−trum thrown upon the table,so as to bring the last quarter of an inch of the read colour upon the stand (after Ref.1).InsideSir FrederickWilliam Herschel (1738–1822)measures infrared light from the sun– artist’s impression (after Ref. 2).Fig.2.The Nobili−Meloni thermopiles:(a)thermopile’s prototype invented by Nobili (ca.1829),(b)incomplete version of the Nobili−−Melloni thermopile (ca.1831).Museo Galileo –Institute and Museum of the History of Science,Piazza dei Giudici 1,50122Florence, Italy (after Ref. 14).Table 1. Milestones in the development of infrared physics and technology (up−dated after Refs. 11 and 12)Year Event1800Discovery of the existence of thermal radiation in the invisible beyond the red by W. HERSCHEL1821Discovery of the thermoelectric effects using an antimony−copper pair by T.J. SEEBECK1830Thermal element for thermal radiation measurement by L. NOBILI1833Thermopile consisting of 10 in−line Sb−Bi thermal pairs by L. NOBILI and M. MELLONI1834Discovery of the PELTIER effect on a current−fed pair of two different conductors by J.C. PELTIER1835Formulation of the hypothesis that light and electromagnetic radiation are of the same nature by A.M. AMPERE1839Solar absorption spectrum of the atmosphere and the role of water vapour by M. MELLONI1840Discovery of the three atmospheric windows by J. HERSCHEL (son of W. HERSCHEL)1857Harmonization of the three thermoelectric effects (SEEBECK, PELTIER, THOMSON) by W. THOMSON (Lord KELVIN)1859Relationship between absorption and emission by G. KIRCHHOFF1864Theory of electromagnetic radiation by J.C. MAXWELL1873Discovery of photoconductive effect in selenium by W. SMITH1876Discovery of photovoltaic effect in selenium (photopiles) by W.G. ADAMS and A.E. DAY1879Empirical relationship between radiation intensity and temperature of a blackbody by J. STEFAN1880Study of absorption characteristics of the atmosphere through a Pt bolometer resistance by S.P. LANGLEY1883Study of transmission characteristics of IR−transparent materials by M. MELLONI1884Thermodynamic derivation of the STEFAN law by L. BOLTZMANN1887Observation of photoelectric effect in the ultraviolet by H. HERTZ1890J. ELSTER and H. GEITEL constructed a photoemissive detector consisted of an alkali−metal cathode1894, 1900Derivation of the wavelength relation of blackbody radiation by J.W. RAYEIGH and W. WIEN1900Discovery of quantum properties of light by M. PLANCK1903Temperature measurements of stars and planets using IR radiometry and spectrometry by W.W. COBLENTZ1905 A. EINSTEIN established the theory of photoelectricity1911R. ROSLING made the first television image tube on the principle of cathode ray tubes constructed by F. Braun in 18971914Application of bolometers for the remote exploration of people and aircrafts ( a man at 200 m and a plane at 1000 m)1917T.W. CASE developed the first infrared photoconductor from substance composed of thallium and sulphur1923W. SCHOTTKY established the theory of dry rectifiers1925V.K. ZWORYKIN made a television image tube (kinescope) then between 1925 and 1933, the first electronic camera with the aid of converter tube (iconoscope)1928Proposal of the idea of the electro−optical converter (including the multistage one) by G. HOLST, J.H. DE BOER, M.C. TEVES, and C.F. VEENEMANS1929L.R. KOHLER made a converter tube with a photocathode (Ag/O/Cs) sensitive in the near infrared1930IR direction finders based on PbS quantum detectors in the wavelength range 1.5–3.0 μm for military applications (GUDDEN, GÖRLICH and KUTSCHER), increased range in World War II to 30 km for ships and 7 km for tanks (3–5 μm)1934First IR image converter1939Development of the first IR display unit in the United States (Sniperscope, Snooperscope)1941R.S. OHL observed the photovoltaic effect shown by a p−n junction in a silicon1942G. EASTMAN (Kodak) offered the first film sensitive to the infrared1947Pneumatically acting, high−detectivity radiation detector by M.J.E. GOLAY1954First imaging cameras based on thermopiles (exposure time of 20 min per image) and on bolometers (4 min)1955Mass production start of IR seeker heads for IR guided rockets in the US (PbS and PbTe detectors, later InSb detectors for Sidewinder rockets)1957Discovery of HgCdTe ternary alloy as infrared detector material by W.D. LAWSON, S. NELSON, and A.S. YOUNG1961Discovery of extrinsic Ge:Hg and its application (linear array) in the first LWIR FLIR systems1965Mass production start of IR cameras for civil applications in Sweden (single−element sensors with optomechanical scanner: AGA Thermografiesystem 660)1970Discovery of charge−couple device (CCD) by W.S. BOYLE and G.E. SMITH1970Production start of IR sensor arrays (monolithic Si−arrays: R.A. SOREF 1968; IR−CCD: 1970; SCHOTTKY diode arrays: F.D.SHEPHERD and A.C. YANG 1973; IR−CMOS: 1980; SPRITE: T. ELIOTT 1981)1975Lunch of national programmes for making spatially high resolution observation systems in the infrared from multielement detectors integrated in a mini cooler (so−called first generation systems): common module (CM) in the United States, thermal imaging commonmodule (TICM) in Great Britain, syteme modulaire termique (SMT) in France1975First In bump hybrid infrared focal plane array1977Discovery of the broken−gap type−II InAs/GaSb superlattices by G.A. SAI−HALASZ, R. TSU, and L. ESAKI1980Development and production of second generation systems [cameras fitted with hybrid HgCdTe(InSb)/Si(readout) FPAs].First demonstration of two−colour back−to−back SWIR GaInAsP detector by J.C. CAMPBELL, A.G. DENTAI, T.P. LEE,and C.A. BURRUS1985Development and mass production of cameras fitted with Schottky diode FPAs (platinum silicide)1990Development and production of quantum well infrared photoconductor (QWIP) hybrid second generation systems1995Production start of IR cameras with uncooled FPAs (focal plane arrays; microbolometer−based and pyroelectric)2000Development and production of third generation infrared systemssitivity was much greater than that of contemporary thermo−piles which were little improved since their use by Melloni. Langley continued to develop his bolometer for the next20 years(400times more sensitive than his first efforts).His latest bolometer could detect the heat from a cow at a dis−tance of quarter of mile [9].From the above information results that at the beginning the development of the IR detectors was connected with ther−mal detectors.The first photon effect,photoconductive ef−fect,was discovered by Smith in1873when he experimented with selenium as an insulator for submarine cables[18].This discovery provided a fertile field of investigation for several decades,though most of the efforts were of doubtful quality. By1927,over1500articles and100patents were listed on photosensitive selenium[19].It should be mentioned that the literature of the early1900’s shows increasing interest in the application of infrared as solution to numerous problems[7].A special contribution of William Coblenz(1873–1962)to infrared radiometry and spectroscopy is marked by huge bib−liography containing hundreds of scientific publications, talks,and abstracts to his credit[20,21].In1915,W.Cob−lentz at the US National Bureau of Standards develops ther−mopile detectors,which he uses to measure the infrared radi−ation from110stars.However,the low sensitivity of early in−frared instruments prevented the detection of other near−IR sources.Work in infrared astronomy remained at a low level until breakthroughs in the development of new,sensitive infrared detectors were achieved in the late1950’s.The principle of photoemission was first demonstrated in1887when Hertz discovered that negatively charged par−ticles were emitted from a conductor if it was irradiated with ultraviolet[22].Further studies revealed that this effect could be produced with visible radiation using an alkali metal electrode [23].Rectifying properties of semiconductor−metal contact were discovered by Ferdinand Braun in1874[24],when he probed a naturally−occurring lead sulphide(galena)crystal with the point of a thin metal wire and noted that current flowed freely in one direction only.Next,Jagadis Chandra Bose demonstrated the use of galena−metal point contact to detect millimetre electromagnetic waves.In1901he filed a U.S patent for a point−contact semiconductor rectifier for detecting radio signals[25].This type of contact called cat’s whisker detector(sometimes also as crystal detector)played serious role in the initial phase of radio development.How−ever,this contact was not used in a radiation detector for the next several decades.Although crystal rectifiers allowed to fabricate simple radio sets,however,by the mid−1920s the predictable performance of vacuum−tubes replaced them in most radio applications.The period between World Wars I and II is marked by the development of photon detectors and image converters and by emergence of infrared spectroscopy as one of the key analytical techniques available to chemists.The image con−verter,developed on the eve of World War II,was of tre−mendous interest to the military because it enabled man to see in the dark.The first IR photoconductor was developed by Theodore W.Case in1917[26].He discovered that a substance com−posed of thallium and sulphur(Tl2S)exhibited photocon−ductivity.Supported by the US Army between1917and 1918,Case adapted these relatively unreliable detectors for use as sensors in an infrared signalling device[27].The pro−totype signalling system,consisting of a60−inch diameter searchlight as the source of radiation and a thallous sulphide detector at the focus of a24−inch diameter paraboloid mir−ror,sent messages18miles through what was described as ‘smoky atmosphere’in1917.However,instability of resis−tance in the presence of light or polarizing voltage,loss of responsivity due to over−exposure to light,high noise,slug−gish response and lack of reproducibility seemed to be inhe−rent weaknesses.Work was discontinued in1918;commu−nication by the detection of infrared radiation appeared dis−tinctly ter Case found that the addition of oxygen greatly enhanced the response [28].The idea of the electro−optical converter,including the multistage one,was proposed by Holst et al.in1928[29]. The first attempt to make the converter was not successful.A working tube consisted of a photocathode in close proxi−mity to a fluorescent screen was made by the authors in 1934 in Philips firm.In about1930,the appearance of the Cs−O−Ag photo−tube,with stable characteristics,to great extent discouraged further development of photoconductive cells until about 1940.The Cs−O−Ag photocathode(also called S−1)elabo−History of infrared detectorsFig.3.Longley’s bolometer(a)composed of two sets of thin plati−num strips(b),a Wheatstone bridge,a battery,and a galvanometer measuring electrical current (after Ref. 15 and 16).rated by Koller and Campbell[30]had a quantum efficiency two orders of magnitude above anything previously studied, and consequently a new era in photoemissive devices was inaugurated[31].In the same year,the Japanese scientists S. Asao and M.Suzuki reported a method for enhancing the sensitivity of silver in the S−1photocathode[32].Consisted of a layer of caesium on oxidized silver,S−1is sensitive with useful response in the near infrared,out to approxi−mately1.2μm,and the visible and ultraviolet region,down to0.3μm.Probably the most significant IR development in the United States during1930’s was the Radio Corporation of America(RCA)IR image tube.During World War II, near−IR(NIR)cathodes were coupled to visible phosphors to provide a NIR image converter.With the establishment of the National Defence Research Committee,the develop−ment of this tube was accelerated.In1942,the tube went into production as the RCA1P25image converter(see Fig.4).This was one of the tubes used during World War II as a part of the”Snooperscope”and”Sniperscope,”which were used for night observation with infrared sources of illumination.Since then various photocathodes have been developed including bialkali photocathodes for the visible region,multialkali photocathodes with high sensitivity ex−tending to the infrared region and alkali halide photocatho−des intended for ultraviolet detection.The early concepts of image intensification were not basically different from those today.However,the early devices suffered from two major deficiencies:poor photo−cathodes and poor ter development of both cathode and coupling technologies changed the image in−tensifier into much more useful device.The concept of image intensification by cascading stages was suggested independently by number of workers.In Great Britain,the work was directed toward proximity focused tubes,while in the United State and in Germany–to electrostatically focused tubes.A history of night vision imaging devices is given by Biberman and Sendall in monograph Electro−Opti−cal Imaging:System Performance and Modelling,SPIE Press,2000[10].The Biberman’s monograph describes the basic trends of infrared optoelectronics development in the USA,Great Britain,France,and Germany.Seven years later Ponomarenko and Filachev completed this monograph writ−ing the book Infrared Techniques and Electro−Optics in Russia:A History1946−2006,SPIE Press,about achieve−ments of IR techniques and electrooptics in the former USSR and Russia [33].In the early1930’s,interest in improved detectors began in Germany[27,34,35].In1933,Edgar W.Kutzscher at the University of Berlin,discovered that lead sulphide(from natural galena found in Sardinia)was photoconductive and had response to about3μm.B.Gudden at the University of Prague used evaporation techniques to develop sensitive PbS films.Work directed by Kutzscher,initially at the Uni−versity of Berlin and later at the Electroacustic Company in Kiel,dealt primarily with the chemical deposition approach to film formation.This work ultimately lead to the fabrica−tion of the most sensitive German detectors.These works were,of course,done under great secrecy and the results were not generally known until after1945.Lead sulphide photoconductors were brought to the manufacturing stage of development in Germany in about1943.Lead sulphide was the first practical infrared detector deployed in a variety of applications during the war.The most notable was the Kiel IV,an airborne IR system that had excellent range and which was produced at Carl Zeiss in Jena under the direction of Werner K. Weihe [6].In1941,Robert J.Cashman improved the technology of thallous sulphide detectors,which led to successful produc−tion[36,37].Cashman,after success with thallous sulphide detectors,concentrated his efforts on lead sulphide detec−tors,which were first produced in the United States at Northwestern University in1944.After World War II Cash−man found that other semiconductors of the lead salt family (PbSe and PbTe)showed promise as infrared detectors[38]. The early detector cells manufactured by Cashman are shown in Fig. 5.Fig.4.The original1P25image converter tube developed by the RCA(a).This device measures115×38mm overall and has7pins.It opera−tion is indicated by the schematic drawing (b).After1945,the wide−ranging German trajectory of research was essentially the direction continued in the USA, Great Britain and Soviet Union under military sponsorship after the war[27,39].Kutzscher’s facilities were captured by the Russians,thus providing the basis for early Soviet detector development.From1946,detector technology was rapidly disseminated to firms such as Mullard Ltd.in Southampton,UK,as part of war reparations,and some−times was accompanied by the valuable tacit knowledge of technical experts.E.W.Kutzscher,for example,was flown to Britain from Kiel after the war,and subsequently had an important influence on American developments when he joined Lockheed Aircraft Co.in Burbank,California as a research scientist.Although the fabrication methods developed for lead salt photoconductors was usually not completely under−stood,their properties are well established and reproducibi−lity could only be achieved after following well−tried reci−pes.Unlike most other semiconductor IR detectors,lead salt photoconductive materials are used in the form of polycrys−talline films approximately1μm thick and with individual crystallites ranging in size from approximately0.1–1.0μm. They are usually prepared by chemical deposition using empirical recipes,which generally yields better uniformity of response and more stable results than the evaporative methods.In order to obtain high−performance detectors, lead chalcogenide films need to be sensitized by oxidation. The oxidation may be carried out by using additives in the deposition bath,by post−deposition heat treatment in the presence of oxygen,or by chemical oxidation of the film. The effect of the oxidant is to introduce sensitizing centres and additional states into the bandgap and thereby increase the lifetime of the photoexcited holes in the p−type material.3.Classification of infrared detectorsObserving a history of the development of the IR detector technology after World War II,many materials have been investigated.A simple theorem,after Norton[40],can be stated:”All physical phenomena in the range of about0.1–1 eV will be proposed for IR detectors”.Among these effects are:thermoelectric power(thermocouples),change in elec−trical conductivity(bolometers),gas expansion(Golay cell), pyroelectricity(pyroelectric detectors),photon drag,Jose−phson effect(Josephson junctions,SQUIDs),internal emis−sion(PtSi Schottky barriers),fundamental absorption(in−trinsic photodetectors),impurity absorption(extrinsic pho−todetectors),low dimensional solids[superlattice(SL), quantum well(QW)and quantum dot(QD)detectors], different type of phase transitions, etc.Figure6gives approximate dates of significant develop−ment efforts for the materials mentioned.The years during World War II saw the origins of modern IR detector tech−nology.Recent success in applying infrared technology to remote sensing problems has been made possible by the successful development of high−performance infrared de−tectors over the last six decades.Photon IR technology com−bined with semiconductor material science,photolithogra−phy technology developed for integrated circuits,and the impetus of Cold War military preparedness have propelled extraordinary advances in IR capabilities within a short time period during the last century [41].The majority of optical detectors can be classified in two broad categories:photon detectors(also called quantum detectors) and thermal detectors.3.1.Photon detectorsIn photon detectors the radiation is absorbed within the material by interaction with electrons either bound to lattice atoms or to impurity atoms or with free electrons.The observed electrical output signal results from the changed electronic energy distribution.The photon detectors show a selective wavelength dependence of response per unit incident radiation power(see Fig.8).They exhibit both a good signal−to−noise performance and a very fast res−ponse.But to achieve this,the photon IR detectors require cryogenic cooling.This is necessary to prevent the thermalHistory of infrared detectorsFig.5.Cashman’s detector cells:(a)Tl2S cell(ca.1943):a grid of two intermeshing comb−line sets of conducting paths were first pro−vided and next the T2S was evaporated over the grid structure;(b) PbS cell(ca.1945)the PbS layer was evaporated on the wall of the tube on which electrical leads had been drawn with aquadag(afterRef. 38).。
租船合同缩写
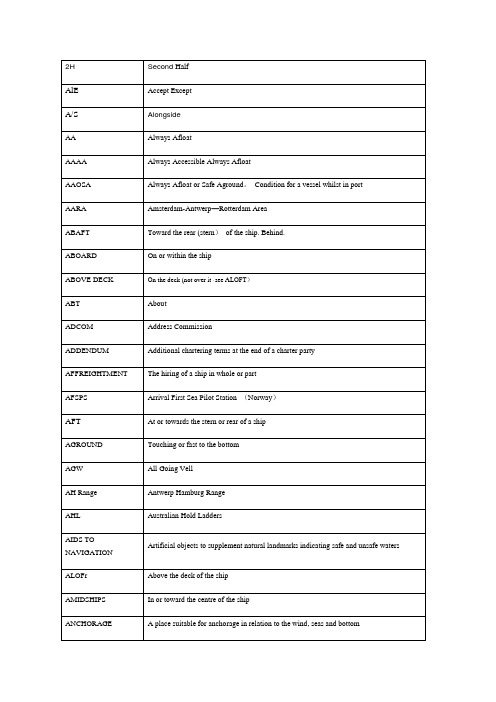
About
ADCOM
Address Commission
ADDENDUM
Additional chartering terms at the end of a charter party
AFFREIGHTMENT
The hiring of a ship in whole or part
AFSPS
AHL
Australian Hold Ladders
AIDS TO NAVIGATION
Artificial objects to supplement natural landmarks indicating safe and unsafe waters
ALOFr
Above the deck of the ship
Avertical partition separating compartments
BUNDLING
This isthe assemblyof pieces of cargo, secured into one manageable unit。
This is relevant to items such asStructuralSteel, Handrails,Stairways etc。
ASTERN
In the hack of the ship. opposite of ahead
ATDNSH[NC
Any Time Day/Night Sundays and Holidays Included
ATHWARTSHIPS
At right angles to the centreline of the ship
AMIDSHIPS
In or toward the centre of the ship
租船合同缩写
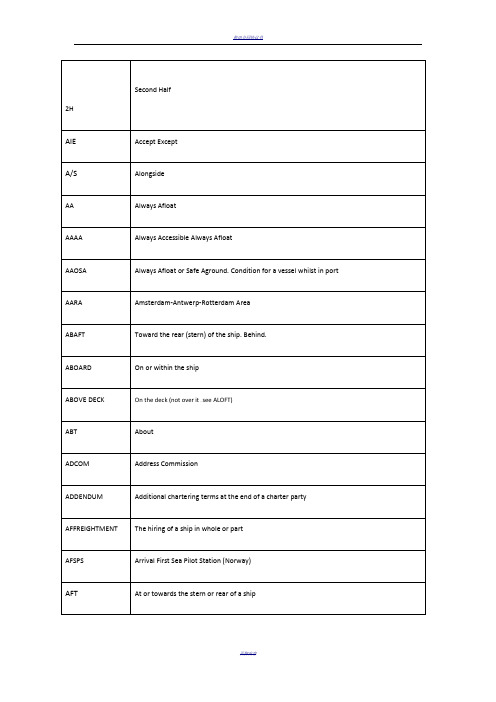
Bareboat Charter— Owners lease a specilic ship and control its technical management and commercial operations only. Charterers take over all responsibility for the operation of the vessel and expenses for the duration.
CHART
A map used by navigators
CHOPT
Charterers Option
CHTRS
Charterers
CIF
Cost. Insurance & Freight. Seller pays all these costs to a nominated port or place of discharge.
AHL
Australian Hold Ladders
AIDS TO NAVIGATION
Artificial objects to supplement natural landmarks indicating safe and unsafe waters
ALOFr
Above the deck of the ship
BN
Booking Note
BOB
Bunker on Board
BOD
Bunker on Delivery
BOFFER
Best Offer
BOW
The forward part of a ship
BOR
Bunker on Re-Delivery
长直平行双导线电感传统计算方法误差分析与修正
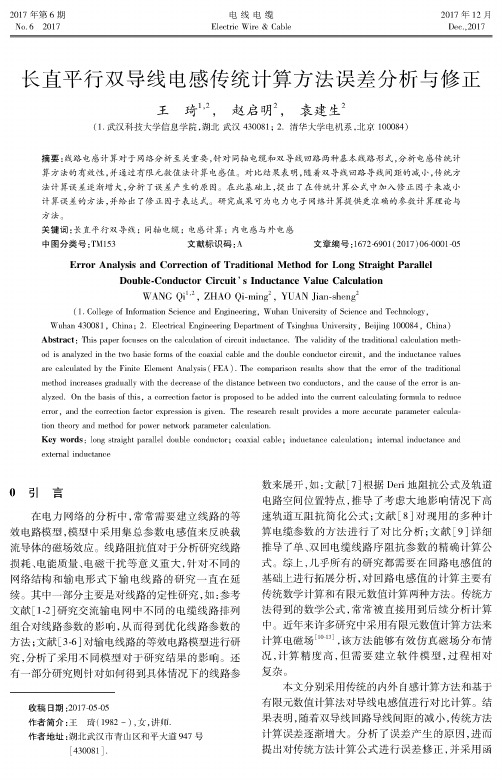
2017年第6期No. 6 2017电线电缆Electric Wire & Cable2017年12月Dec.,2017长直平行双导线电感传统计算方法误差分析与修正王琦1>2,赵启明2,袁建生2(1.武汉科技大学信息学院,湖北武汉43〇〇Sl; 2.清华大学电机系,北京1〇〇〇84)摘要:线路电感计算对于网络分析至关重要,针对同轴电缆和双导线回路两种基本线路形式,分析电感传统计 算方法的有效性,并通过有限元数值法计算电感值。
对比结果表明,随着双导线回路导线间距的减小,传统方 法计算误差逐渐增大,分析了误差产生的原因。
在此基础上,提出了在传统计算公式中加入修正因子来减小 计算误差的方法,并给出了修正因子表达式。
研究成果可为电力电子网络计算提供更准确的参数计算理论与 方法。
关键词:长直平行双导线;同轴电缆;电感计算;内电感与外电感中图分类号:TM153文献标识码:A文章编号:1672~6901(2017)06~0001-05Error Analysis and Correction of Traditional Method for Long Straight ParallelDouble-Conductor Circuit' s Inductance Value CalculationWANG Qi 1'2, ZHAO Qi -ming 2, YUAN Jian -sheng 2(1. College of Information Science and Engineering, Wuhan University of Science and Technology,Wuhan 430081, China ; 2. Electrical Engineering Department of Tsinghua University, Beijing 100084, China) Abstract : This paper focuses on the calculation of circuit inductance. The validity of the traditional calculation method is analyzed in the two basic forms of the coaxial cable and the double conductor circuit, and the inductance values are calculated by the Finite Element Analysis ( FEA). The comparison results show that the error of the traditional method increases gradually with the decrease of the distance between two conductors, and the cause of the error is analyzed. On the basis of this, a correction factor is proposed to be added into the current calculating formula to reduce error, and the correction factor expression is given. The research result provides a more accurate parameter calculation theory and method for power network parameter calculation.Key words : long straight parallel double conductor ; coaxial cable ; inductance calculation ; internal inductance and external inductance 〇引言在电力网络的分析中,常常需要建立线路的等 效电路模型,模型中采用集总参数电感值来反映载 流导体的磁场效应。
electrical current 英文解释
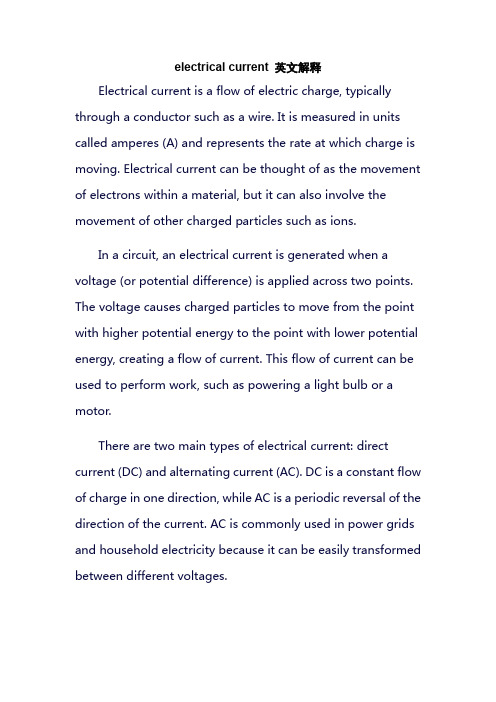
electrical current 英文解释Electrical current is a flow of electric charge, typically through a conductor such as a wire. It is measured in units called amperes (A) and represents the rate at which charge is moving. Electrical current can be thought of as the movement of electrons within a material, but it can also involve the movement of other charged particles such as ions.In a circuit, an electrical current is generated when a voltage (or potential difference) is applied across two points. The voltage causes charged particles to move from the point with higher potential energy to the point with lower potential energy, creating a flow of current. This flow of current can be used to perform work, such as powering a light bulb or a motor.There are two main types of electrical current: direct current (DC) and alternating current (AC). DC is a constant flow of charge in one direction, while AC is a periodic reversal of the direction of the current. AC is commonly used in power grids and household electricity because it can be easily transformed between different voltages.Electrical current is also closely related to magnetism, as described by Ampere's law and Faraday's law of electromagnetic induction. These relationships are exploited in many electrical and electronic devices, including motors, generators, and transformers.。
3B SCIENTIFIC 高压管说明书
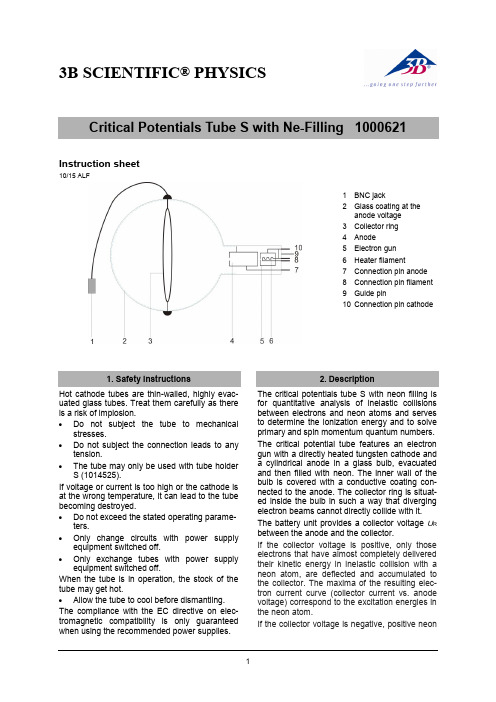
3B SCIENTIFIC ®PHYSICSInstruction sheet10/15 ALF1 BNC jack2 Glass coating at theanode voltage 3 Collector ring 4 Anode5 Electron gun6 Heater filament7 Connection pin anode 8 Connection pin filament 9 Guide pin10 Connection pin cathodeHot cathode tubes are thin-walled, highly evac-uated glass tubes. Treat them carefully as there is a risk of implosion.∙ Do not subject the tube to mechanicalstresses.∙ Do not subject the connection leads to anytension.∙ The tube may only be used with tube holderS (1014525).If voltage or current is too high or the cathode is at the wrong temperature, it can lead to the tube becoming destroyed.∙ Do not exceed the stated operating parame-ters.∙ Only change circuits with power supplyequipment switched off.∙ Only exchange tubes with power supplyequipment switched off.When the tube is in operation, the stock of the tube may get hot.∙ Allow the tube to cool before dismantling. The compliance with the EC directive on elec-tromagnetic compatibility isonly guaranteed when using the recommended power supplies.The critical potentials tube S with neon filling is for quantitative analysis of inelastic collisions between electrons and neon atoms and serves to determine the ionization energy and to solve primary and spin momentum quantum numbers. The critical potential tube features an electron gun with a directly heated tungsten cathode and a cylindrical anode in a glass bulb, evacuated and then filled with neon. The inner wall of the bulb is covered with a conductive coating con-nected to the anode. The collector ring is situat-ed inside the bulb in such a way that diverging electron beams cannot directly collide with it. The battery unit provides a collector voltage U R between the anode and the collector.If the collector voltage is positive, only those electrons that have almost completely delivered their kinetic energy in inelastic collision with a neon atom, are deflected and accumulated to the collector. The maxima of the resulting elec-tron current curve (collector current vs. anode voltage) correspond to the excitation energies in the neon atom.If the collector voltage is negative, positive neonions are deflected and collected to the collector. The ionization energy of the neon atom can be read off from the course of the resulting ion cur-rent curve (collector current vs. anode voltage).1 Critical potentials tube S with Ne filling1 Battery unit (battery not included)1 Shielding1 ManualGas filling: NeonFilament voltage: U F≤ 7 V DCAnode voltage: U A≤ 60 VAnode current: I A≤ 10 mACollector voltage: U R = 1,5 VCollector current: I R≤ 200 pAGlass bulb: 130 mm diam. approx Length of tube: 260 mm approx.Critical potentials of neon:2p53s1: 16.6 eV2p53p1: 18.4 eV2p54s1: 19.7 eV2p54p1: 20.3 eV2p54d1: 20.6 eVIonization: 21.6 eVFor operating the tube:1 Tube holder S 1014525 1 Control Unit for Critical Potential Tubes (115 V or 230 V) 1000633 / 1008506 1 DC-Power Supply, 0–20 V(115 V or 230 V) 1003311 / 1003312 or1 Power Supply Unit for Franck-Hertz Experiment (115 V or 230 V) 1012819 / 1012818 For making measurements:1 Analogue Oscilloscope 2x30 MHz 10027272 HF Patch Cords, BNC/4 mm Plug 1002748 or1 3B NET log TM(115 V or 230 V) 1000539 / 1000540 1 3B NET lab TM1000544 1 computer1 Battery AA 1.5 V1 Set of 15 Safety Experiment Leads 10028436.1 Setting up the tube in the tube holder∙The tube should not be mounted or removed unless all power supplies are disconnected. ∙Press tube gently into the stock of the holder and push until the pins are fully inserted. Take note of the unique position of the guide pin.6.2 Removing the tube from the tube holder ∙Allow the tube to cool before dismantling.∙To remove the tube, apply pressure on the guide pin until the pins loosen, then pull out the tube.Determine the critical potentials of a neon atom7.1 General notesThe experiment set-up with the critical potential tube is highly sensitive to sources of electromag-netic interference. (computers, fluorescent lights). ∙Select a location for the experiment where electromagnetic interference can be avoided.Circuit schematicUAR: Collector ringA: AnodeC: Cathode7.2 Experiment set-up with the control unitfor critical potential tubes∙Insert the tube into the tube holder. Provision of heater voltage U F:∙Connect the sockets F3 of the tube holder to the positive terminal of the DC power supply and F4 to the negative terminal. (see Fig. 1) Provision of accelerating voltage U A:∙Connect socket C5 of the tube holder to the negative terminal of the output V A on the control unit and to the negative terminal of the DC power supply.∙Connect socket A1 to the positive terminal of the output V A on the control unit.Provision of collector voltage U R:∙Put the shielding over the tube and slot it into the groove on the tube holder so that the tube is completely enclosed by shielding. Then connect the control unit by means of the ground socket.∙Connect the lead from the collector ring to the BNC input socket of the control unit.∙Clip the battery into the battery holder with the correct polarity.∙The positive pole of the output V A should be connected to the negative pole of the 1.5 V battery.∙Connect the positive terminal of the 1.5 V battery to an earth socket on the control unit. Note:The collector voltage is now positive as required for recording the curve for the electron current. In this case, the current measurement values are negative.In order to halve the collector voltage, the positive pole of the control unit can be connected to termi-nal B of the battery holder.To record the ion current curve, the battery polarity is reversed in order to obtain a negative collector voltage. The current measurements are then posi-tive.7.2.1 Procedure with 3B NET log TM∙At the output V A of the control unit, set the minimum voltage to about 10 V and the maximum voltage to about 35 V, by using the 3B NET log™ unit to measure the voltages (smaller by a factor of 1000) between socket 3 and earth and that between socket 4 and earth. Alternatively, the voltages can be set up with the help of a multimeter.∙Connect the 3B NET log™ unit to the computer.∙Connect the output “Fast 1” from the control unit to input A of the 3B NET log™ unit and the output “Fast 2” to input B. (See Fig. 2)∙Switch on the 3B NET log™ unit and start the 3B NET lab™ program on the computer.∙Select the “Measurement lab” function and open a new data record.∙Select analogue inputs A and B and DC voltage mode (VDC), setting the measurement ranges to 200 mV for A and2 V for B.∙Enter the formula I= -667 * “Input_B“ (unit pA).∙Set the following parameters: Measurement interval = 50 µs, Measurement duration =0.05 s, Mode = Recorder.∙Set triggering on the input A with rising edge (20%).∙On the DC power supply, set the heater voltage to 3.5 V.∙Start the graph-plotting of the experimental data.∙Set up the graph with “relative time t in s” on the x-axis and the quantity I on the y-axis.∙Repeat the measurements with slightly higher heater voltages and vary the minimum and maximum accelerating voltages U A to find the optimum graph.∙In the spectrum, identify the 2p53S1 peak at16.6 eV and determine its position t1 on thetime axis.∙Identify the ionization threshold at 21.6 eV and determine its position t2on the time axis.∙Enter a new formula for the quantity E defined as 16.6 + 5 * (t - t1)/(t2 - t1) with the unit eV; in this expression enter the numerical values for t1and t2in s determined as above.Set up a graph with the quantity E on the x-axis and the quantity I on the y-axis. (See Fig. 3).In order to display the ion current curve, reverse the polarity of the collector voltage.Fig. 1 Experiment set-up with the control unit for critical potential tubesHERTZ TUBE CONSOLE60V A+1OUT34200 mV!Fig. 2 Connection of 3B NET log TM to the control unit for critical potential tubesFig. 3: Determination of critical potentials for neon atoms (curve recorded using 3B NET log TM). Excitation ener-gies at 16,6 eV (2p53s1-level), 18,4 eV (2p53p1 level), 19,7 eV (2p54s1 level), 20,3 eV (2p54p1 level) and 20,6 eV (2p54d1 level) and ionization energy at 21,6 eV7.2.2 Procedure with an oscilloscope∙Connect output Fast1 on the control unit to Channel 1 (X deflection) on the oscilloscope and output Fast 2 to Channel 2 (Y deflec-tion). (See Fig. 4)∙Set the minimum voltage output V A on the control unit to approximately 10 V and the maximum voltage to about 35 V. Use a mul-timeter to measure the voltage between socket 3 and ground or socket 4 and ground, which will be a factor of 1000 times smaller.∙On the DC power supply, set the heater voltage to 3.5 V. Oscilloscope settings:Channel 1: 50 mV/divChannel 2: 0.2 V/divTime-base: 5 msTrigger on Channel 1∙Vary the heating voltage, the upper and lower limits of the accelerating voltage and the os-cilloscope parameters until the best curve can be seen.∙In order to display the ionization curve, re-verse the polarity of the collector voltage.Fig. 4 Connection of an oscilloscope to the control unit for critical potential tubes7.3 Experiment set-up with the control unitfor the Franck-Hertz experiment∙Insert the tube into the tube holder. Provision of heater voltage U F:∙Connect socket F3 on the tube holder to socket F on the control unit for the Franck-Hertz experiment and connect socket F4 to socket K. (refer to Fig. 5)Provision of accelerating voltage U A:∙Connect socket C5 on the tube holder to socket K on the control unit and socket A1 to socket A. Note:The collector voltage U R is supplied internally from the Franck-Hertz experiment control unit. It can be varied between 0 V and 12 V and is positive when the set opposing (decelerating) voltage is shown as negative. The values measured for the electron current are then negative.∙Put the shielding over the tube and slot it into the groove on the tube holder so that the tube is completely enclosed by shielding.Then connect it to the ground socket of the control unit for the Franck-Hertz experiment. ∙Connect the collector ring to the BNC input on the control unit.Fig. 5 Experiment set-up with the control unit for the Franck-Hertz experiment7.3.1 Procedure with 3B NET log TM∙Connect the 3B NET log TM unit to the control unit for the Franck-Hertz experiment (see Fig. 6). Connect the Ux output of the control unit to input A of the 3B NET log TM unit and output Uy to input B.∙Set the control unit to ramp mode and con-figure a minimum voltage of about 10 V, witha maximum voltage of about 35 V.∙Select a heater voltage of some 3.5 V and a collector voltage of around -1.5 V.∙Set up the 3B NET log TM interface, the 3B NET lab TM computer program and the measurement recording as described in sec-tion 7.2.1.∙Vary the heating voltage, the upper and lower limits of the accelerating voltage and the gain until the best optimal can be seen.∙In order to display the ion current curve, reverse the polarity of the collector voltage. 7.3.2 Procedure with an oscilloscope∙Connect the Ux output of the control unit to Channel 1 (X deflection) of the oscilloscope and output Uy to Channel 2 (Y deflection) (see Fig. 7).∙Set the control unit to ramp mode and con-figure a minimum voltage of about 10 V, witha maximum voltage of about 35 V.∙Select a heater voltage of some 3.5 V and a collector voltage of around -1.5 V. Oscilloscope settings:Channel 1: 50 mV/divChannel 2: 0.2 V/divTime-base: 5 msTrigger on Channel 1∙Vary the heating voltage, the upper and lower limits of the accelerating voltage and the os-cilloscope parameters until the best curve can be seen.∙In order to display the ion current curve, reverse the polarity of the collector voltage.Fig. 6 Connection of 3B NET log TM to the control unit for the Franck-Hertz experimentFig. 7 Connection of oscilloscope to the control unit for the Franck-Hertz experiment7.3.3 Calibration of measurement curve∙Set the heater voltage to 3.5 V and collector voltage to -1.5 V; set the lower limit for the accelerating voltage to 0 V and the upper limit to 60 V. Turn up the gain.The oscilloscope screen shows a curve which has a weakly defined structure in three places. The first of these structures are of interest here. To empha-sise these places, carry out the following proce-dure.∙Reduce the upper limit of the accelerating voltage down to about 35 V.This zooms in on the measurement curve and the structures become clearer.∙In order to display the curve even bigger, raise either the gain or the heater voltage.You may need to change the oscilloscope settings.∙Raise the upper limit of the accelerating voltage (to about 10 V) until the curve starts at the edge of the first peak. You may need to increase the gain to display the structures better.∙Reduce the upper limit of the accelerating voltage (to about 25 V) until the curve ends at the point where ionization begins.The range of the curve in which the critical po-tentials lie is now displayed within clearly de-fined limits on the oscilloscope screen and the critical potentials themselves can specifically be identified.3B Scientific GmbH ▪ Rudorffweg 8 ▪ 21031 Hamburg ▪ Germany ▪ 。
3B S CIENTIFIC 演示多功能多尺度电压电流计说明书
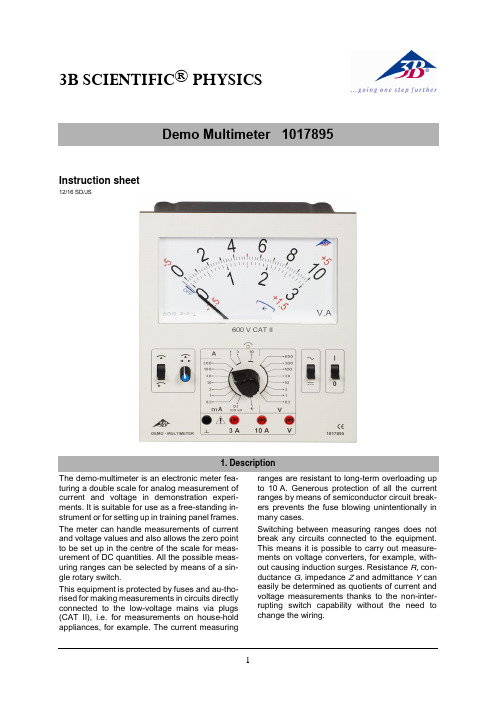
3B SCIENTIFIC ® PHYSICSInstruction sheet12/16 SD/JSThe demo-multimeter is an electronic meter fea-turing a double scale for analog measurement of current and voltage in demonstration experi-ments. It is suitable for use as a free-standing in-strument or for setting up in training panel frames. The meter can handle measurements of current and voltage values and also allows the zero point to be set up in the centre of the scale for meas-urement of DC quantities. All the possible meas-uring ranges can be selected by means of a sin-gle rotary switch.This equipment is protected by fuses and au-tho-rised for making measurements in circuits directly connected to the low-voltage mains via plugs (CAT II), i.e. for measurements on house-hold appliances, for example. The current measuringranges are resistant to long-term overloading up to 10 A. Generous protection of all the current ranges by means of semiconductor circuit break-ers prevents the fuse blowing unintentionally in many cases.Switching between measuring ranges does not break any circuits connected to the equipment. This means it is possible to carry out measure-ments on voltage converters, for example, with-out causing induction surges. Resistance R , con-ductance G, impedance Z and admittance Ycan easily be determined as quotients of current and voltage measurements thanks to the non-inter-rupting switch capability without the need to change the wiring.The demo multimeter conforms to safety regula-tions for electrical measurement, control and la-boratory equipment, as specified in DIN EN 61010-1, protection class 2 and to measuring ca-tegory CAT II for up to 600 V. The nominal volt-age between the phase conductors and the neu-tral for voltage and current measurements (in cir-cuits directly connected to mains electricity) must not exceed 600 V in order to conform to CAT II. The meter is intended for measurements within its measuring ranges and in a measuring environ-ment as described in detail in the course of this manual. Safe operation of the multimeter is gua-ranteed if it is solely used as specified. Safety can-not be guaranteed, however, if the multimeter is used incorrectly or handled without due care and attention. In order to avoid serious injury due to current or voltage shocks, the following safety in-structions are to be observed at all times.The multimeter may only be used by persons who are able to recognise the risks of contact and take due precautions to avoid them. Voltages in ex-cess of 33 V AC (RMS) or 70 V DC are to be re-garded as actively dangerous if the current, charge or energy stored should exceed certain values (see DIN EN 61010-1).∙Carefully read the instruction manual before using the multimeter and obey the instruc-tions therein.∙The multimeter may only be used in a dry, dust-free environment with no risk of explo-sions occurring.The assumption needs to be made that unfore-seen voltages may be present in the vincinity of objects being measured (e.g. faulty equipment). ∙Before using the multimeter, check the hou-sing and measuring leads for damage and if there should be any malfunctions or visible damage, the multimeter is not to be used.Pay specific attention to the insulation for the measuring sockets.∙The multimeter may not be used to make measurement on circuits which exhibit co-rona discharge (high voltage).∙Particular care is to be taken when making measurements on high-frequency circuits where dangerous voltages may arise due to superimposition of components.∙The authorised measuring range is not to be exceeded. If measurements are made when the magnitude of the variable is unknown, al-ways select a large measuring range before shifting down to lower ones. ∙Make very sure that the voltage value be-tween the measured contact and earth or be-tween the ground socket and the measure-ment socket does not exceed 600 V.∙Before using the analogue multimeter to check that a voltage source is not exhibiting any actual voltage, check that the meter is working properly by selecting the battery test function.∙When measuring current, make sure the electricity is turned off before the analogue multimeter is connected into the circuit.∙When making measurements, always con-nect the ground lead first. Disconnect the sig-nal measurement lead before unplugging the ground.∙Turn off the multimeter before opening the casing, disconnect the power to the circuit and the measuring leads from the multimeter. If measurements are made where there are any risks of coming into contact with electricity, a sec-ond person is to be informed.∙The demo-multimeter should not be stored, set up or operated within reach of children. ∙When the multimeter is used by teenagers, trainees etc., a suitable person should super-vise to ensure the equipment is used safely. ∙If measurements are to be made where volt-ages exceed 33 V AC (RMS) or 70 V DC, be especially careful and only use safety experi-ment leads.Measuring categories according to DIN EN 61010-1.CAT I or unstipulated: Approved for measure-ments in circuits which are not directly connected to the low voltage mains grid (e.g. batteries). CAT II: Approved for measurements in circuits which are directly connected, by a mains lead and plug for instance, to the low voltage mains grid (e.g. household or office appliance and lab equipment).CAT III: Approved for measurements in circuits which are part of a building’s wiring installation (e.g. stationary consumers, distribution terminals, appliances connected directly to the distribution box).CAT IV: Approved for measurements in circuits which are directly connected to the source of the low voltage mains (e.g. electricity meters, main service feed, primary excess voltage protection).Scales: 0 … 10, linear0 … 3, linearScale length: 160 mmPointer deflection: 0…90°Electricalzero-point offset: in all DC rangesMeasurements:Voltage ranges: 0.1/ 0.3/ 1/ 3/ 10/ 30/100/ 300/ 600 V AC/DC Current ranges: 0.1/ 0.3/ 1/ 3/ 10/ 30/100/ 300 mA AC/DC1/ 3/ 10 A AC/DCInput resistance: 1 MΩ AC/DCVoltage drop whenmeasuring current: 100 mV approx. AC/DCReference conditions:Ambient temperature: 23 °COperating alignment: VerticalSignal form: Sine (1% max. discrep-ancy)Peak factor:Frequency range: 40 Hz … 50 Hz … 5 kHzAccuracy (at reference conditions):DC quantities: Class 2DC withzero-point offset: Class 5AC quantities: Class 3Extended frequency range (class 10):3 – 600 V: 40 Hz … 50 Hz … 40 kHz0,3 – 1 V: 40 Hz … 50 Hz … 10 kHz0,3 – 3000 mA: 40 Hz … 50 Hz … 40 kHz10 A: 40 Hz … 50 Hz … 40 kHz Resistance, conductance, impedance, admit-tanceThese quantities can be determined by forming various quotients involving “simultaneous meas-urements” of current and voltage.R = U / I: below 1 mΩ … above 10 MΩS = I / U: below 1 µS … above 30 SZ = U / I: below 1 mΩ … above 10 MΩ,40 Hz … 40 kHzY = I / U: below 1 µS … above 30 S,40 Hz … 40 kHz Voltage ranges: 600 V long-term in allvoltage ranges Current ranges: 10 A of long-term load-ing in 3-A and 10-ArangesElectrical safety:Safety specifications: EN 61010-1 Measuring category: CAT II: 600 V Contamination level: 2Protection type: IP20Connectors: 4-mm safety socketsProtection:Fuses: 2x FF 10 A/600 V(10 x 38 mm) Breaking capacity: at least 10 kA3B order number: 5008564Power supply:Battery: 1x 1.5 V, AA IEC LR6 Automatic cut-off after: 45 min ± 10 minElectromagnetic compatibility: Interference emission: EN 55011:2009 Interference resistance: EN 61326-1:2013Operating conditions:Ambient temperature: 5 °C ... 23 °C … 40°C Storage temperature: -20 … 70°CRelative humidity: <85% with no conden-sationGeneral data:Shock test: max. 147 m/s² Height: 297 mm Dimensions: 259 x 297 x 125 mm3 Weight: 1,7 kg approx.1 Display2 Slotted screw for zero calibration 3Toggle switch 1Zero point centre / left4 Calibration trimmer for settingcentre zero point5 Rotary switch to select the mea-surement range6 Ground socket7 Current measurement socket forup to 3 A8 Current measurement socket forup to 10 A9 Voltage measurement socket 10 Toggle switch 2AC / DC voltage measurements 11 Power switch4.2 Rear12 Cover plate for battery andfuses13 Rating plate 14 Fuse diagram 15 Lower edge 16 FeetDisplayFrontRearEarth symbol∙ Set up the demo multimeter vertically.∙ Do not connect measuring leads to begin with.∙ ∙The needle will point to the zero point of the scale on the left. If it does not, the amount of charge of the battery should be checked.7.1 To switch on:∙7.2 Checking battery charge: ∙ Turn on the demo-multimeter. ∙ Disconnect all measuring leads.∙ Set the toggle switch 2 to .∙Set the rotary switch to.If the battery is sufficiently charged, the needle to the following range indication,If this is not the case, the battery will7.3 Zero point calibration:∙ Turn on the demo-multimeter. ∙ Turn the rotary switch to 600 V.∙ Connect the common/ground socket and thevoltage measurement socket together by means of a short connecting lead.∙ Turn the zero-point trimmer screw to adjustthe zero point as needed.7.4 Zero point calibration for centre zeropoint:For measurements of DC current and voltage, the zero point of the scale can be moved to the centre of the dial. For this purpose the scales are la-belled with red numbers..∙ Turn on the demo-multimeter. ∙ Disconnect all measuring leads.∙ Set the toggle switch 2 to . ∙ ∙Use the zero-point trimmer to line up the nee-dle precisely in the centre of the dial (red di-vision).7.5 To switch off:∙ Set the power switch to .∙Set the four-way switch to .When the meter is turned off, the needle points to.7.6 If a measurement is interrupted by batterycut-out:After 45 minutes of use, the multimeter is auto-matically shut off and the needle will then point to. To switch back on:∙ Set the power switch of the multimeter to offand then use it to turn the meter back on.∙If measurements are made when the magni-tude of the variable is unknown, always selecta large measuring range before shifting downto lower ones.∙Connect the terminal at the lower potential to the common/ground socket.∙Connect the common/ground lead first and only then the signal lead.8.1 DC currents up to 3 A:∙Set the toggle switch 2 to .∙Alternatively, if measurements are to be∙Select the required current measuring range to a range measured in mA or A.8.2 AC currents up to 3 A:∙∙Select the required current measuring range to a range measured in mA or A.∙Set up a measuring range of 10 A.∙Connect the terminal at the lower potential to the common/ground socket.∙Connect the common/ground lead first and only then the signal lead.9.1 DC currents up to 10 A:∙Set the toggle switch 2 to .∙Alternatively, if measurements are to be9.2 AC currents up to 10 A:∙∙If measurements are made when the magni-tude of the variable is unknown, always select a large measuring range before shifting down to lower ones.∙Connect the common/ground lead first and only then the signal lead.10.1 DC voltages up to 600 V:∙ Set the toggle switch 2 to .∙Alternatively, if measurements are to be∙ Select the required measuring range to a range measured in V.∙For measurements of voltage up to 100 mV, set the rotary switch to the range 0.1 mA/100 mV.10.2 AC voltages up to 600 V:∙∙ Select the required measuring range to a range measured in V.∙For measurements of voltage up to 100 mV, set the rotary switch to the range 0.1 mA/100 mV.11.1 DC voltages and currents:∙ Set the toggle switch 2 to .∙Use the rotary switch to set the desired volt-age measuring range and read off the meas-urement.∙Set a suitable current measuring range and read off the measurement.11.2 AC voltages and currents:∙∙Use the rotary switch to set the desired volt-age measuring range and read off the meas-urement.∙Set a suitable current measuring range and read off the measurement.The demo-multimeter has a compartment which houses both the battery and fuses and which is accessible at the rear once its cover is opened. One fuse each is provided for the 3 A and 10 A sockets:FF10 A/600 V, breaking capacity: at least 10 kA (3B order number: 5008564)The polarity is indicated by plus and minus signs inside the fuse holder compartment. A mechani-cal system ensures the battery makes no contact if it is inserted the wrong way round.Battery and fuse compartment12.1 Battery testing:Batteries which are discharged and have not been used for a while may leak.12.2 Changing the battery:∙Remove the cover at the rear.∙Replace flat batteries with 1.5-V alkaline bat-teries of size AA IEC LR6.∙Place the negative pole of the battery on thespring.∙Close the cover again afterwards.12.3 Changing fuses:∙Remove the cover at the rear.∙Check the fuses.∙Blown fuses should be replaced with ones ofthe same rating.∙Close the cover again afterwards.∙For cleaning, use a soft cloth, slightly mois-tened with alcohol, or a brush.Electrostatic charging of the display window canaffect the measurements under certain circum-stances:∙To remove such charge, use a soft clothslightly soaked in alcohol or a paint brush.Dirt or moisture in the measurement sockets canaffect readings.∙Shake out any dirt that may be in the meas-urement sockets.∙Soak a new swab with isopropyl alcohol andwork around the inside of each measurementsocket.∙The packaging should be disposed of at localrecycling points.Should you need to dis-pose of the equipment it-self, never throw it away innormal domestic waste. Ifbeing used in privatehouseholds it can be dis-posed of at the local publicwaste disposal authority.∙Comply with the applicable regulations for thedisposal of electrical equipment.∙Do not dispose of the batteries in the regularhousehold garbage. Follow the applicable le-gal regulations (UK: Waste Batteries and Ac-cumulators Regulations, EU: 2006/66/EC).3B Scientific GmbH ▪ Rudorffweg 8 ▪ 21031 Hamburg ▪ Germany ▪ 。
D.C.Circuits
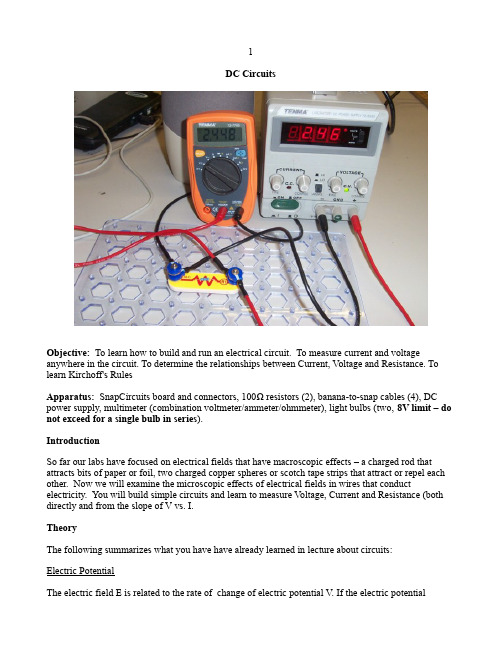
DC CircuitsObjective: To learn how to build and run an electrical circuit. To measure current and voltage anywhere in the circuit. To determine the relationships between Current, V oltage and Resistance. To learn Kirchoff's RulesApparatus: SnapCircuits board and connectors, 100Ω resistors (2), banana-to-snap cables (4), DC power supply, multimeter (combination voltmeter/ammeter/ohmmeter), light bulbs (two, 8V limit – do not exceed for a single bulb in series).IntroductionSo far our labs have focused on electrical fields that have macroscopic effects – a charged rod that attracts bits of paper or foil, two charged copper spheres or scotch tape strips that attract or repel each other. Now we will examine the microscopic effects of electrical fields in wires that conduct electricity. You will build simple circuits and learn to measure V oltage, Current and Resistance (both directly and from the slope of V vs. I.TheoryThe following summarizes what you have have already learned in lecture about circuits:Electric PotentialThe electric field E is related to the rate of change of electric potential V. If the electric potentialchanges by the amount V, the electric field in the direction of the displacement is:E=− V sCurrentCurrent I is defined as the flow of charge Q per until time:I= Q tPowerPower P is the rate of energy flow per unit time through a circuit or circuit element:P=VI=I2ROhm's LawFor many a material, the current I through it is proportional to the potential difference across it, with the resistance R being the constant of proportionality, as well as the slope of the V vs. I graph:V = IRMaterials that behave this law are known as ohmic materials; those that do not are non-ohmic.The power that is dissipated through a resistor also obeys P=I2R.Kirchoff's Junction RuleThe current entering any point in a circuit must equal the current leaving that point. This ensures that there is no charge buildup anywhere, which would violate conservation of charge and energy:A junction is where roads or paths meet orconnect. In the junction on the right, the current I1splits up into I2 and I3. So that there is no excessbuildup of charge or current in the circuit, thiscondition must be fulfilled:I1 = I2 + I3. or I1 - I2 - I3 = 0Kirchoff's Loop RuleThe algebraic sum of all potential differences around any closed loop in a circuit is zero:Starting from the battery V b and proceedingcounterclockwise, the gains or losses in potentialsare:+V b – IR1 – IR2 = 0ProcedureYou will build a series of circuits using SnapCircuit circuit elements and connectors, which will be connected to a DC power supply. A DC (Direct Current) power supply supplies a steady potential difference (voltage), as opposed to the AC (Alternation Current) power which comes out of an electrical outlet at home or in lab. A typical flashlight battery is another example of a DC power source.Circuit elements, including light bulbs and power supplies/batteries, have two terminals: plus (+) and minus (-). Current enters through one and and leaves through the other. So a closed circuit (as in the circuit containing two resistor and one battery, above) should be one continuous, closed loop (or series of loops).On your power supply, there is a green Power button. Make sure that, before you push it, the “Coarse” and “Fine” Current knobs are set to the middle position, and that the “Coarse” V oltage knob is set to the minimum position. After you press the Power button, you can slowly adjust the V oltage with the two V oltage knows. Do not exceed 8V on the power supply if you are using a single light bulb in series with it. The voltage (or current) is displayed on the red LED display on the face of the power supply, with a lever that lets you select V oltage or Current. Remember, this is the voltage (or current) reading through the power supply and not necessarily at every point in the circuit.IMPORTANT: A voltmeter must be connected in parallel with the circuit element across which you are measuring the voltage; that is, the current forks out to enter both the element and the voltmeter, then recombines after it has passed through both. In contrast, an ammeter must be connected in series with the circuit element through which you are measuring the current; this means that the ammeter must be directly in-line with the circuit element. In the labs, we will be using measuring devices called multimeters, which can serve as a voltmeter, ammeter or ohmmeter. If you are using the multimeter as an ohmmeter, you should not have a power supply or battery in the circuit – the only thing in between the ohmmeter's terminals should be the circuit element (resistor or bulb).function dial is set to V--, which measuresDC voltage; you will not use V~, whichmeasures AC voltage, in this lab. The nextfunction is Ω which measures resistance.The middle setting is for diodes, which youwill not use, and the next three – μA, mAand A – measure current.You will use two cables, banana-to-button,to connect the multimeter to various partsof the circuits that you will build, or circuitelements. On the multimeter, one of thesecables stays on the COM jack at bottomleft. The other cable is to be plugged intothe VΩmA jack on bottom center ifmeasuring voltage or resistance, or the 10Ajack on bottom left if measuring current.Remember that the multimeter cables areto be connected to a circuit in parallel ifmeasuring voltage. If you're measuringcurrent, the cables are to be eonnect to thecircuit in series!Record all drawings, observations and calculations in the hand-in sheet and submit.Activity 0Connect the cables of an ohmmeter (select this function on your multimeter) to the terminals of a 100 Ohm resistor and confirm that the resistor's rating is approximately correct..Activity 1The power supply has a selectable current-limiter; this is a feature that limits the current, and hence the power (from P=I2R) in the circuit. This prevents the device being powered (light bulb, amplifier, etc.) from overloaded. Activate the current limiter by doing the following:a. With the green Power button OFF, turn all Current and V oltage knobs to their minimum position(fully counterclockwise). Then press the Power button ON.b. SHORT the two lead wires (+ and -) coming out of the power supply by connecting the button-snap ends to each other.c. With the two lead wires still snapped together, rotate the COARSE V oltage knob about 1/8 turn clockwise from its minimum position and set the V olts/Amps selector switch on the LED read-out to AMPS.d. Adjust the Current knobs until the read-out indicates 0.150 Amps (this corresponds to roughly 8V when the bulb is fully lit, the bulb's maximum rating). This power supply will make sure that this value is not exceeded. You can now proceed to hook up the light bulb in the next step.Activity 2Build a simple circuit where a flashlight bulb is powered by a DC power supply. Slowly raise the voltage to between 7 and 8 V olts. Remember, do not exceed 8V on the power supply if you are using a single light bulb in series with it. Draw the circuit in pictorial form (like a street artist, so that your TA can see exactly how you connected things when he/she grades your lab report), and also draw its representation in schematic form (using symbols for resistor, battery, etc).Activity 3Measure the voltage across the bulb. Do this two different ways. (Hint – you have more than one multimeter at your disposal.) Remember how a voltmeter is connected to a circuit.Activity 4Measure the current through the light bulb. Do this two different ways. Remember how an ammeter is connected to a circuit.Activity 5Using only the power supply's built-in multimeter, vary the voltage across the light bulb from zero to the maximum rating of the light bulb at 11 evenly-spaced values of voltage. At each value, record the current (this involves the mere flip of the Current/V oltage switch on the power supply's meter). Activity 6-9Repeat Activities 2-5 above, but this time for a 100 Ohm resistor; do not use any light bulbs. For the single resistor, use the current limiter to keep the current to under 0.07 A (this corresponds to the rating of the resistor – about 0.5 W).Plot V vs. I for both bulb and resistor; you can use Logger Pro if you wish (recommended). Is there a difference between the shapes of their graphs?For the resistor, find a value for the slope of the V vs. I graph. How does this value compare to theresistance value as labeled on the resistor? Is the resistor an ohmic device? Is the bulb an ohmic device?Activity 10Connect two100Ω resistors in series and connect to the power supply. Predict (calculate) the voltage across each one and the current through each one. Then actually measure these values using multimeter(s). How close (give a percentage error) were your predicted values to your measured ones? Draw the complete circuit and indicate the current through and voltage drop across each circuit element (including the power supply). Are Kirchoff's Rules obeyed (do the numbers add up)?Activity 11Connect two 100Ω resistors in parallel and connect to the power supply. If you have trouble visualizing what it would look like on your SnapCircuits board, draw it out in schematic form first. Predict (calculate) the voltage across each one and the current through each one. Then actually measure these values using multimeter(s). How close (give a percentage error) were your predicted values to your measured ones? Draw the complete circuit and indicate the current through and voltage drop across each circuit element (including the power supply). Are Kirchoff's Rules obeyed (do the numbers add up)?Assessment and Presentation (Hand-in Sheet/Lab Notebook)After entering results from Activities 1-10 above in the hand-in sheet, answer the following questions: 1. Resistance is often a function of temperature. How does the temperature of the light bulb influence is resistance?2. Christmas Tree light bulbs are connected in parallel, not series. Can you think of a practical reason for this?3. Connect a light bulb in series with a 100 Ohm resistor and connect to a power supply. Are you able to make the bulb light up? DO NOT EXCEED 8V! If not, explain why.。
Gravity duals for non-relativistic CFTs
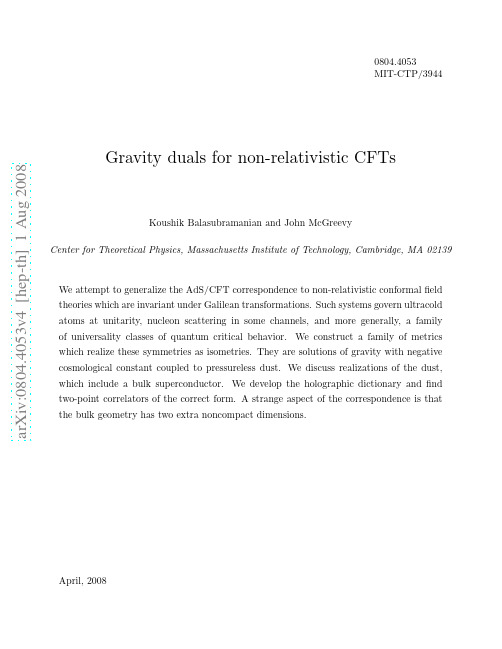
a r X i v :0804.4053v 4 [h e p -t h ] 1 A u g 20080804.4053MIT-CTP/3944Gravity duals for non-relativistic CFTs Koushik Balasubramanian and John McGreevy Center for Theoretical Physics,Massachusetts Institute of Technology,Cambridge,MA 02139We attempt to generalize the AdS/CFT correspondence to non-relativistic conformal field theories which are invariant under Galilean transformations.Such systems govern ultracold atoms at unitarity,nucleon scattering in some channels,and more generally,a family of universality classes of quantum critical behavior.We construct a family of metrics which realize these symmetries as isometries.They are solutions of gravity with negative cosmological constant coupled to pressureless dust.We discuss realizations of the dust,which include a bulk superconductor.We develop the holographic dictionary and find two-point correlators of the correct form.A strange aspect of the correspondence is that the bulk geometry has two extra noncompact dimensions.April,20081.IntroductionMany attempts have been made to use the AdS/CFT correspondence[1,2,3]to study systems realizable in a laboratory.One does not yet have a holographic dual matching the precise microscopic details of any such system and is therefore led to try to match the universality class of the system.In general,physics in the far infrared is described by a(sometimes trivial)fixed point of the renormalization group.It has been argued that the associated zero-temperature conformalfield theory(CFT)controls a swath of the finite-temperature phase diagram,namely the region in which the temperature is the only important scale,and no dimensionful couplings have turned on(see e.g.[4]).In trying to use gauge/string duality to study such questions,it seems urgent,then,to match the symmetries to those of the sytem of interest.The AdS/CFT correspondence so far gives an effective description of relativistic con-formalfield theories at strong coupling.Not many of these are accessible experimentally.However,there are many non-relativistic conformalfield theories which govern phys-ical systems.Such examples arise in condensed matter physics[4],atomic physics[5], and nuclear physics[6].In thefirst situation,these are called‘quantum critical points’; this term refers to a second-order phase transition that is reached not by varying the temperature,but by varying some coupling constants at zero temperature.A particularly interesting example where exquisite experimental control is possible is the case of cold fermionic atoms at unitarity(e.g.[5]and references therein and thereto). These are scale invariant in the following sense.The interactions are tuned(by manipu-lating the energy of a two-body boundstate to threshold)to make the scattering length infinite(hence the system is strongly coupled).The material is dilute enough that the effective range of the potential can be treated as zero.While the mass of the atoms is a dimensionful quantity,the dependence of the energy and other physical quantities on that mass is determined by the symmetry algebra.A recent paper studying the constraints from Galilean conformal symmetry on such systems is[7](other recent theory references include[8]).In this brief note,we set out tofind a bulk dual of non-relativistic CFTs,analogous to the AdS gravity description of relativistic CFTs,at strong coupling.We approach this question by considering the algebra of generators of the non-relativistic conformal group, which appears in[7](related work includes[9]).We seek a solution of string theory(in its low-energy gravity approximation)whose asymptotic boundary symmetry group is not the1Poincar´e symmetry group(i.e.Lorentz and translations),but rather Galilean invariance and translations.We also demand a non-relativistic version of scale invariance and,when possible,special conformal transformations.Clearly we will have to introduce some background energy density tofind such a solu-tion(i.e.it will not be just a solution of gravity with a cosmological constant).Holograph-ically,this is because the theories we are trying to describe arise in general(perhaps not always)from relativistic microscopic theories(not necessarily conformal)upon by adding in some background of Stuff(whichfixes a preferred reference frame)and then taking some limit focussing on excitations with small velocities in this frame.An analogy which may be useful is the following.The plane-wave symmetry group is a contraction of the AdS isometry group;the plane wave solution arises from AdS in considering a limit focussing on the worldline of a fast-moving particle.So it might be possible to take some limit of AdS analogous to the plane wave limit tofind our solution. The limit involved would be like the Penrose limit,but replacing the single particle world-line with a uniform background number density.We leave such a derivation from a more microscopic system for the future and proceed to guess the end result.Scale invariance can be realized in non-relativistic theories in a number of ways.One freedom is the relative scale dimension of time and space,called the‘dynamical exponent’z(see e.g.[10,11]).The familiar Schr¨o dinger case has z=2,and the conformal algebra for this case is described in[7].Fixed points with z=2can be Galilean invariant,too. We willfind a metric with this symmetry group as its isometry group for each value of z.1 To be specific,the relevant algebra is:[M ij,N]=[M ij,D]=0,[M ij,P k]=i(δik P j−δjk P i),[M ij,K k]=i(δik K j−δjk K i) [M ij,M kl]=i(δik M jk−δjk M il+δil M kj−δjl M ki)[P i,P j]=[K i,K j]=0,[K i,P j]=iδij N,[D,P i]=iP i,[D,K i]=(1−z)iK i [H,N]=[H,P i]=[H,M ij]=0,[H,K i]=−iP i,[D,H]=ziH,[D,N]=i(2−z)N.(1.1) Here i,j=1..d label the spatial dimensions.M ij generate spatial rotations,and thefirst two lines just state the properties of the various generators under spatial rotations.P i are momenta,K i generate Galilean boosts,N is a conserved rest mass or particle number,andD is the dilatation operator.That the particle number can be conserved is a consequence of the possibility of absence of antiparticles in a non-relativistic theory2.For the special case z=2,there is an additional special conformal generator C which enjoys the algebra:[M ij,C]=0,[K i,C]=0,[D,C]=−2iC,[H,C]=−iD.The metrics wefind are also invariant under the combined operations of time-reversal and charge conjugation.In the next section we write down a metric with this isometry group.3It is sourced by a stress tensor describing a negative cosmological constant plus dust.After demonstrating the action of the isometry group,we provide answers to the important question:why doesn’t the dust collapse due to the gravitational attraction?In section three,we generalize the AdS/CFT dictionary to extract information about thefield theory dual,including anomalous dimensions and Green’s functions.In section four,we highlight some of the many open questions raised by this construction.2.GeometryAfter all this talk,here,finally is the metric:ds2=L2 −dt2r2+dr22That is to say,the dualfield theories that we are describing must not have particle production. It is of course possible for non-relativistic theories to nevertheless contain both particles and antiparticles,as indeed do the systems under study in[12],which,unlike ours,are invariant under t→−t.3Earlier work on geometric realizations of the Schr¨o dinger group include[13]and references therein,wherein related metrics are called Bargmann-Einstein structures.4Note added:In the case d=2,z=3,this metric has appeared previously as the near-horizon limit of D3-branes in the presence of lightlike tensorfields[14];its causal structure was studied in [15].The sources present in those solutions do not preserve the rotations M ij.32.1.SymmetriesWe shall show that the isometries of the above metric comprise the d+1-dimensional non-relativistic dilatation group with algebra(1.1).It is clear that the above metric is invariant under translations in x,t and under rotations of x.It is also invariant under the scale transformationx′=λx,t′=λz t,r′=λr,ξ′=λ2−zξ.It is invariant under the Galilean boostx′= x− v t(2.2)if we assign the following transformation to the coordinateξ:ξ′=ξ+11+ct ,t′=t1+ct,ξ′=ξ+c1+ct.To understand the transformation rule forξ,let us define the transformations T1and T2, such thatT1:(x,t)→ x t ,T2:(x,t)→(x,t+c);(2.4) T1is an inversion and T2is a time-translation.The special conformal transformation S can be written as S=T1T2T1.One can check that the vectorfields generating these isometries have Lie brackets satisfying the algebra(1.1).5The only non-obvious identification is that the rest mass is generated by N=i∂ξ.Note that although the metric is not invariant under simple time reversal,t→−t,it is invariant under the combined operationt→−t,ξ→−ξ,(2.5) which,given the interpretation of theξ-momentum as rest mass,can be interpreted as the composition,CT,of charge conjugation and time-reversal.2.2.Einstein’s equationsThe stress tensor sourcing the metric in equation(2.1)consists of a negative cosmo-logical constant6Λ=−10plus a pressureless‘dust’,with constant density E:T ab=−Λg ab−Eδ0aδ0b g00.For d=3,wefind that the density is E= 2z2+z−3 L−2.In the following subsection,we consider what matter can produce this stress tensor. Our answers to this question do not affect our later calculations,except to suggest what fields should be present in the bulk,and to demonstrate that the metric(2.1)is physically sensible.2.3.DustWe observe that because of the non-stationary form of the metric,the stress tensor for an electricfield in the radial direction F rt has only a00component.Maxwell’s equation in the bulk,coupled to a background current j a is1∂a √grα−2.(2.7)(α−2)(α−d−2)The current and gaugefield are therefore related by the London equation j a=m2A A a, with m2A=z(z+d)g −12|DΦ|2−V |Φ|2with D aΦ≡(∂a+ieA a)Φ,with a Mexican-hat potentialV |Φ|2 =g |Φ|2−v2 2+Λproduces the correct dust stress tensor for arbitrary g,as long as e2v2=m2A=z(z+d)2z+dL4.It is tempting to relate the phase ofΦto that of the boundary wavefunction.We hope in the future to use this theory withfinite g to describe the vortex lattice formed by rotating the cold-atom superfluids.The fact that the metric(2.1)is sourced by a reasonable class of physical systems with stable ground states suggests that it need have no intrinsic instabilities.3.CorrespondenceConsider a scalarfield in the background(2.1),with actionS=−1g ∂µφ∂νφgµν+m2φ2 ,Here d d+3x≡d d x dtdξdr.The usual argument[2]that h xy(k z)satisfies the same equation of motion as a massless scalar goes through,and so we can take the m=0case as a proxy for this component of the metricfluctuations.In the metric(2.1),we have√√ggµν∂νφ)−m2φ= r d+3∂r 1r d+1∂r +r2(2lω+ k2)+r4−2z l2+m2 fω, k,l(r)=0.Tofind the generalization of the gauge/gravity dictionary to this case,wefirst study asymptotic solutions of this equation near the boundary.Writing f∝r∆,wefind for z≤2:∆±=1+d 1+d8Our metric is therefore a solution of the same system studied in thefirst and last references in[17],with very different asymptotics.6In the case z>2,there is no scaling solution near the boundary;theξ-momentum domi-nates the boundary behavior,and the solutions vary with r faster than any power of r.It would be interesting to understand a possible meaning of the asymptotic solutions which do arise,and to interpret the critical nature of z=2in terms of the boundary theory.As usual,these exponents∆±can be interpreted as scaling dimensions of spin-zero boundary operators in the boundary theory.We look forward to extending this analysis to thefluc-tuations offields of other spin.Instead we will proceed to compute correlation functions of these scalar operators.3.1.CorrelatorsFor definiteness,we focus on the familiar,critical z=2case with d=3spatial dimensions.In that case,the momentum in theξ-direction simply adds to the mass of the bulk scalar,i.e.they appear in the combination l2+m2.In this case,the wave equation becomes −r6∂r 159For general d,with z=2,the solution isf(r)=Ar1+dω, k,l1+dTo compute correlators,we introduce a cutoffnear the boundary at r=ǫ,and nor-malize fκ(ǫ)=1(so A=ǫ−5/2Kν(κǫ)−1).The usual prescription goes through,leaving an on-shell bulk action of the formS[φ0]=1gg rrφ(X)∂rφ(X) r=ǫwhere X∈{ x,t,ξ}are the coordinates on the boundary.This evaluates toS[φ0]=1gg rr fκ(r)∂r fκ(r)=√2Kν(κr) |r=ǫ. Using the expansion for small xKν(x)≃2ν−1Γ(ν)x−ν 1+...− xΓ(1+ν)and ignoring contact terms,this isF(κ,ǫ)=−Γ(1−ν)2ǫ5 κǫΓ(ν)14ν(in the caseφ=h xy,O=T xy).We defer a more careful treatment of holographic renormalization to future work.Fourier transforming to position space,this isO1(x,t)O2(0,0) ∝Γ(1−ν)|ǫ2t|∆e−ilx2/2|t|where we used translation invariance to put the second operator at( x,t)=( 0,0),θisa step function,and∆i,j are the scaling dimensions of the operators that we found in(3.1).This is the form one expects for the correlators in a Galilean-invariant conformal field theory in d spatial dimensions with dynamical exponent2.It will be interesting to extend this calculation to other values of z.84.DiscussionIn discussions of AdS/CFT,one often hears that the CFT‘lives at the boundary’of the bulk spacetime.The spacetime(2.1)is conformal to a pp-wave spacetime,and hence has a boundary which is one dimensional–for z>1,g tt grows faster than the other components at small r.While one might be tempted to speculate that this means that the dualfield theory is one dimensional,there is no clear evidence for this statement(in the usual case,only the UV of thefield theory can really be said to live at the boundary of the AdS space),and indeed we have nevertheless used it to compute correlators which look like those of a d-dimensional nonrelativisticfield theory.Perhaps the most mysterious aspect of this new correspondence is not of too few dimensions,but of too many10.Theξdirection is an additional noncompact dimension of the bulk geometry besides the usual radial direction,which seems to be associated to the conserved rest mass11.We have computed correlators atfixed l;this is reasonable since the theory is non-relativistic and so a sector with definite l can be closed(unlike in a relativistic theory where these sectors unavoidably mix by production of back-to-back particles and antiparticles).At the special value of the dynamical exponent z=2,varying l affects not just the units in which energy is measured,but the values of the critical exponents(see equation(3.1)).We would like to think of these solutions as scaling limits which zoom in on small fluctuations about particular states of relativistic theories(possibly CFTs),where a pre-ferred rest frame isfixed by some density of Stuff.It would be nice to see our metric arise as such a contraction of an asymptotically AdS solution.Such an identification may help find thefinite-temperature geometry.A word of caution in this direction is in order:at large R-charge but low temperature, there is an instability in supersymmetric theories to Bose condensation,because there are inevitably scalarfields that carry R-charge.A system with symmetries under which only fermions are charged(such as[21])could be useful,and would seem more likely to be related to cold fermions away from thefixed point.Many questions remain.We have computed the correlator T xy T xy .Does this plus the dynamical exponent completely determine the T T correlator,or are there independent central charges in the non-relativistic theory?One interesting observable,which has been measured as well as computed numerically for cold-fermion systems is the analog of the famous3/4in the N=4SYM theory,namely the ratio of the energy density at unitarity to the energy density of the corresponding free theory(ξin[22]and[8],proportional toβin[23]).12We will need to learn to compute the analog of the ADM mass for spacetimes with the asymptopium of(2.1).Once thefinite-temperature solution with these asymptotics is found,there will be many more interesting things to compute.Of course,it will be nice to check the universality ofη12We thank Krishna Rajagopal for teaching us about this.References[1]J.Maldacena,“The large N limit of superconformalfield theories and supergrav-ity,”Adv.Theor.Math.Phys.2,231(1998)[Int.J.Theor.Phys.38,1113(1999)] [arXiv:hep-th/9711200].[2]S.S.Gubser,I.R.Klebanov and A.M.Polyakov,“Gauge theory correlators fromnon-critical string theory,”Phys.Lett.B428,105(1998)[arXiv:hep-th/9802109]. [3] E.Witten,“Anti-de Sitter space and holography,”Adv.Theor.Math.Phys.2,253(1998)[arXiv:hep-th/9802150].[4]S.Sachdev,Quantum Phase Transitions,Cambridge,1999.[5]K.M.O’Hara et.al.,“Observation of a strongly interacting degenerate Fermi gas ofatoms,”Science298,2179(2002);C.A.Regal,M.Greiner,and D.S.Jin,“Obser-vation of Resonance Condensation of Fermionic Atom Pairs,”Phys.Rev.Lett.92, 040403(2004);M.Bartenstein et.al.,“Crossover from a molecular Bose-Einstein con-densate to a degenerate Fermi gas,”Phys.Rev.Lett.92,120401(2004);M.Zwierlein et.al.,“Condensation of Pairs of Fermionic Atoms Near a Feshbach Resonance,”Phys.Rev.Lett.92,120403(2004);J.Kinast et.al.,“Evidence for superfluidity in a res-onantly interacting Fermi gas,”Phys.Rev.Lett.92,150402(2004);T.Bourdel et.al.,“Experimental study of the BEC-BCS crossover region in lithium6,”Phys.Rev.Lett.93,050401(2004).[6]T.Mehen,I.W.Stewart and M.B.Wise,“Conformal invariance for non-relativisticfield theory,”Phys.Lett.B474,145(2000)[arXiv:hep-th/9910025].[7]Y.Nishida and D.T.Son,“Nonrelativistic conformalfield theories,”Phys.Rev.D76,086004(2007)[arXiv:0706.3746[hep-th]].[8]Y.Nishida and D.T.Son,“ǫexpansion for a Fermi gas at infinite scatteringlength,”Phys.Rev.Lett.97,050403(2006)[arXiv:cond-mat/0604500];F.Werner and Y.Castin,“The unitary gas in an isotropic harmonic trap:symmetry properties and applications,”Phys.Rev.A74,053604(2006)[cond-mat/0607821];P.Nikoli´c and S.Sachdev,“Renormalization groupfixed points,universal phase diagram,and1/N expansion for quantum liquids with interactions near the unitarity limit,”Phys.Rev.A75,033608(2007)[arXiv:cond-mat/0609106];M.Y.Veillette,D.E.Sheehy,and L Radzihovsky,“Large-N expansion for unitary superfluid Fermi gases,”Phys.Rev.A75,043614(2007)[arXiv:cond-mat/0610798];Y.Nishida,D.T.Son and S.Tan, Phys.Rev.Lett.100,090405(2008)[arXiv:0711.1562[cond-mat.other]];T.Mehen, arXiv:0712.0867[cond-mat.other].[9]U.Niederer,“The maximal kinematical invariance group of the free Schr¨o dinger equa-tion,”Helv.Phys.Acta45,802(1972);C.R.Hagen,”Scale and Conformal Transfor-mations in Galilean-Covariant Field Theory,”Phys.Rev.D5,377(1972);R.Jackiwand S.Pi,“Finite and Infinite Symmetries in(2+1)-Dimensional Field Theory,”hep-th/9206092;[10]P.C.Hohenberg and B.I.Halperin,Rev.Mod.Phys.49436(1977).[11] E.Ardonne,P.Fendley and E.Fradkin,“Topological order and conformal quantumcritical points,”Annals Phys.310,493(2004)[arXiv:cond-mat/0311466].[12]S.Kachru,X.Liu,and M.Mulligan,“Holography and dynamical critical phenomena,”to appear.[13] C.Duval,G.W.Gibbons and P.Horvathy,“Celestial Mechanics,Conformal Struc-tures,and Gravitational Waves,”Phys.Rev.D43,3907(1991)[arXiv:hep-th/0512188].[14]M.Alishahiha,Y.Oz and J.G.Russo,“Supergravity and light-like non-commutativity,”JHEP0009,002(2000)[arXiv:hep-th/0007215].[15]V.E.Hubeny,M.Rangamani and S.F.Ross,“Causal structures and holography,”JHEP0507,037(2005)[arXiv:hep-th/0504034].[16]J.Erlich,E.Katz,D.T.Son and M.A.Stephanov,“QCD and a holographic modelof hadrons,”Phys.Rev.Lett.95,261602(2005)[arXiv:hep-ph/0501128].[17]S.S.Gubser,“Breaking an Abelian gauge symmetry near a black hole horizon,”arXiv:0801.2977[hep-th];S.S.Gubser,“Colorful horizons with charge in anti-de Sitter space,”arXiv:0803.3483[hep-th];S.A.Hartnoll,C.P.Herzog and G.T.Horowitz,“Building an AdS/CFT superconductor,”arXiv:0803.3295[hep-th].[18] B.Freivogel,Y.Sekino,L.Susskind and C.P.Yeh,“A holographic framework foreternal inflation,”Phys.Rev.D74,086003(2006)[arXiv:hep-th/0606204].[19] E.Ponton and E.Poppitz,“Gravity localization on string-like defects in codimen-sion two and the AdS/CFT correspondence,”JHEP0102,042(2001)[arXiv:hep-th/0012033].[20]S.Weinberg,“Dynamics at infinite momentum,”Phys.Rev.150,1313(1966).[21]T.Sakai and S.Sugimoto,“Low energy hadron physics in holographic QCD,”Prog.Theor.Phys.113,843(2005)[arXiv:hep-th/0412141].[22]T.Schafer,“What atomic liquids can teach us about quark liquids,”Prog.Theor.Phys.Suppl.168,303(2007)[arXiv:hep-ph/0703141].[23]J.T.Stewart et.al.,Phys.Rev.Lett.97,220406(2006)[arXiv:cond-mat/0607776].[24] A.Buchel and J.T.Liu,“Universality of the shear viscosity in supergravity,”Phys.Rev.Lett.93,090602(2004)[arXiv:hep-th/0311175];P.Kovtun,D.T.Son andA.O.Starinets,“Viscosity in strongly interacting quantumfield theories from blackhole physics,”Phys.Rev.Lett.94,111601(2005)[arXiv:hep-th/0405231].[25]M.Van Raamsdonk,arXiv:0802.3224[hep-th].[26]M.Chertkov,I.Kolokolov,and M.Vergassola,“Inverse versus Direct Cascades inTurbulent Advection,”Phys.Rev.Lett.80,512(1998).[27] D.Son,arXiv:0804.3972[hep-th].。
国家电网公司专业技术人员电力英语水平考试题库答案(第二版)完整
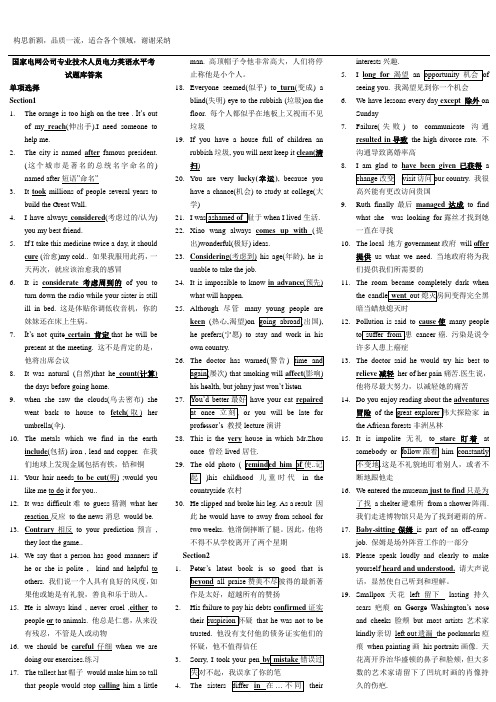
国家电网公司专业技术人员电力英语水平考试题库答案单项选择Section11.The orange is too high on the tree . It’s outof my reach(伸出手).I need someone tohelp me.2.The city is named after famous president.(这个城市是著名的总统名字命名的)named after短语”命名”3.It took millions of people several years tobuild the Great Wall.4.I have always considered(考虑过的/认为)you my best friend.5.If I take this medicine twice a day, it shouldcure (治愈)my cold.. 如果我服用此药,一天两次,就应该治愈我的感冒6.It is considerate 考虑周到的of you toturn down the radio while your sister is stillill in bed. 这是体贴你调低收音机,你的妹妹还在床上生病。
7.It’s not quite certain 肯定that he will bepresent at the meeting. 这不是肯定的是,他将出席会议8.It was natural (自然)that he count(计算)the days before going home.9.when she saw the clouds(乌去密布) shewent back to house to fetch(取) herumbrella(伞).10.The metals which we find in the earthinclude(包括) iron , lead and copper. 在我们地球上发现金属包括有铁,铅和铜11.Your hair needs to be cut(剪) ;would youlike me to do it for you..12.It was difficult难to guess猜测what herreaction反应to the news消息would be.13.Contrary相反to your prediction预言,they lost the game..14.We say that a person has good manners ifhe or she is polite , kind and helpful toothers. 我们说一个人具有良好的风度,如果他或她是有礼貌,善良和乐于助人。
AnalyzingtheSepicConverter
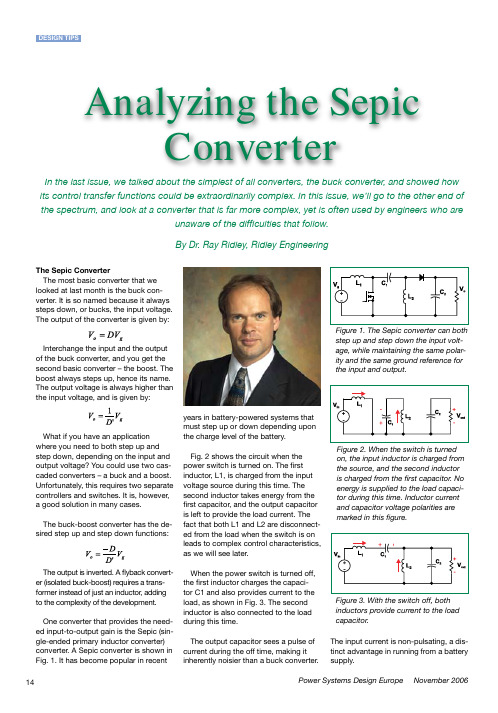
Power Systems Design Europe November 200614By Dr. Ray Ridley, Ridley EngineeringThe Sepic ConverterThe most basic converter that we looked at last month is the buck con-verter. It is so named because it always steps down, or bucks, the input voltage. The output of the converter is given by:Interchange the input and the output of the buck converter, and you get the second basic converter – the boost. The boost always steps up, hence its name. The output voltage is always higher than the input voltage, and is given by:What if you have an application where you need to both step up and step down, depending on the input and output voltage? You could use two cas-caded converters – a buck and a boost. Unfortunately, this requires two separate controllers and switches. It is, however, a good solution in many cases. The buck-boost converter has the de-sired step up and step down functions:The output is inverted. A flyback convert -er (isolated buck-boost) requires a trans-former instead of just an inductor , adding to the complexity of the development. One converter that provides the need-ed input-to-output gain is the Sepic (sin-gle-ended primary inductor converter) converter. A Sepic converter is shown in Fig. 1. It has become popular in recent years in battery-powered systems that must step up or down depending upon the charge level of the battery.Fig. 2 shows the circuit when the power switch is turned on. The first inductor, L1, is charged from the input voltage source during this time. The second inductor takes energy from the first capacitor, and the output capacitor is left to provide the load current. The fact that both L1 and L2 are disconnect-ed from the load when the switch is on leads to complex control characteristics, as we will see later.When the power switch is turned off, the first inductor charges the capaci -tor C1 and also provides current to the load, as shown in Fig. 3. The second inductor is also connected to the load during this time.The output capacitor sees a pulse of current during the off time, making it inherently noisier than a buck converter.The input current is non-pulsating, a dis-tinct advantage in running from a battery supply.ConverterIn the last issue, we talked about the simplest of all converters, the buck converter, and showed how its control transfer functions could be extraordinarily complex. In this issue, we’ll go to the other end of the spectrum, and look at a converter that is far more complex, yet is often used by engineers who areunaware of the difficulties that follow.Figure 1. The Sepic converter can both step up and step down the input volt-age, while maintaining the same polar-Figure 2. When the switch is turned on, the input inductor is charged from the source, and the second inductor is charged from the first capacitor. No energy is supplied to the load capaci-tor during this time. Inductor current and capacitor voltage polarities are marked in this figure.Figure 3. With the switch off, both inductors provide current to the load capacitor.网w w .r d 2.Power Systems Design Europe November 200616The PWM Switch Model in the Sepic ConverterThe best way to analyze both the AC and DC characteristics of the Sepic converter is by using the PWM switch model, developed by Dr. Vatché Vor-périan in 1986. Some minor circuit ma-nipulations are first needed to reveal the location of the switch model, and this is Proper small-signal analysis of the Sepic converter is a difficult analytical task, only made practical by advanced circuit analysis techniques originally de-veloped by Dr. David Middlebrook and continued by Vorpérian. [1]If you’re going to build a Sepic, as a minimum, you need to understand the control characteristics. Fortunately, Vorpérian’s work is now available for this converter, and you can download the complete analysis notes .[2]The simplified analysis of the Sepic converter, derived in detail in [2], ignores parasitic resistances of the inductors and capacitors, and yields the following result for the control-to-output transfer function:Whereshown in Fig. 4.First, capacitor C1 is moved to the bottom branch of the converter. Then, inductor L2 is pulled over to the left, keeping its ends connected to the same nodes of the circuit. This reveals the PWM switch model of the converter, with its active, passive, and common ports, allowing us to use well-estab-lished analysis results for this converter. For more background on the PWM switch model, the text book “FastAnalytical Techniques for Electrical and Electronic Circuits” [1] is highly recom-mended.DC Analysis of the Sepic Converter Fig. 5 shows the equivalent circuit of the Sepic converter with the DC portion of the PWM switch model in place. The DC model is just a 1:D transformer. We replace the inductors with short circuits, and the capacitors with open circuits for the DC analysis. You can, if you like, include any parasitic resistances in the model [2], but that’s beyond the scope of this article.After the circuit is manipulated as shown in the figure, we can write the KVL equation around the outer loop of the converter:Rearranging gives:And the DC gain is given by:Here we see the ability of the con-verter to step up or down, with a gain of 1 when D=0.5. Unlike the buck-boostand Cuk converters, the output is not inverted.AC Analysis of the Sepic Converter Y ou won’t find a complete analysis of the Sepic converter anywhere in printed literature. What you will find are applica -tion notes with comments like, “the Sepic is not well-understood.” Despite the lack of documentation for the converter, engi-neers continue to use it when applicable.Figure 5. For DC analysis, the smallsignal sources are set to zero, induc-tors become short circuits, and capaci-tors become open circuits. After thecircuit is redrawn, it is a trivial matter towrite KVL around the outer loop of the circuit to solve for the conversion gain of the converter.Figure 6. The small-signal AC sources are included in the switch model, and we can either solve the analysis by hand, or use PSpice to plot desired transfer functions. The hand analysis is crucial for symbolic expressions and design equations.Figure 4. In order to take advantage of Vorpérian’s PWM switch model, the circuit elements must first be rear -ranged. The function of the original topology is retained when the capaci-tor is moved, and the second inductor is redrawn.(w w w .r d 211.c om )Power Systems Design Europe November 200618er? There are several possibilities. First, the dynamic and step load requirements on the system may be very benign, with no reason to design a loop with high bandwidth. This allows the loop gain to be reduced below 0 dB before the extreme phase delay of the second resonance.Secondly, in many practical cases, the parasitic resistances of the circuit movethe RHP zeros to the left half plane, greatly reducing the phase delay. This can also be done with the addition of damping networks to the power stage, a topic beyond the scope of this article.Thirdly, some engineers do not builda proper Sepic. In some applicationnotes, the two inductors are wound on a single toroidal core, which provides almost unity coupling between the two. In this case, the circuit no longer works as a proper Sepic. Don’t fall into this design trap - the circuit will be far fromoptimum.Additional Reading [1] “Fast Analytical Techniques for Electrical and Electronic Circuits”,Vatché Vorpérian, Cambridge University Press 2002. ISBN 0 521 62442 8.[2] . Click on Articles and Sepic Analysis Notes.As you can see from these expres-sions, the “simplified” analysis is any -thing but simple. Including the para-sitic resistances greatly complicates the analysis, but may be necessary for worst-case analysis of the Sepic converter. The analysis of this converter involves the use of the powerful extra element theorem, and Vorpérian’s book on circuit analysis techniques. [1]In addition to the inevitable fourth-order denominator of the Sepic, the most important features to note in the control transfer function are the terms in the numerator. The first term is a single right-half-plane (RHP) zero. Right-half-plane zeros are a result of converters where the response to an increased duty cycle is to initially decrease the output voltage.When the power switch is turned on, the first inductor is disconnected from the load, and this directly gives rise to the first-order RHP zero. Notice that the expres-sion only depends on the input inductor, L1, the load resistor, R, and the duty cycle. The complex RHP zeros arise from the fact that turning on the switch disconnects the second inductor from the load. These zeros will actually move with the values of parasitic resistors in the circuit, so careful analysis of your converter is needed to ensure stability under all conditions. PSpice Modeling of the Sepic ConverterThe analytical solution above does not include all of the parasitic circuit ele-ments. As you will see from [2], there is a prodigious amount of work to be done even without the resistances.We can also use PSpice to help un-derstand the Sepic better. Fig. 7 shows the circuit model for a specific numeri -cal application of the Sepic, and it in-cludes resistances which will affect the stability of the converter, sometimes in dramatic ways.The PSpice file listing can be down-loaded from [2] so you can reproduce these results to analyze your own Sepic converter.Fig. 8 shows the result of the PSpice analysis. The two resonant frequen-cies predicted by the hand analysis can clearly be seen in the transfer functionplot. What is remarkable is the extreme amount of phase shift after the second resonance. This is caused by the delay of the second pair of poles, and theadditional delay of the complex RHPzeros. The total phase delay throughthe converter is an astonishing 630degrees. Controlling this converter at a frequency beyond the second reso-nance is impossible.SummaryThe Sepic converter definitely hassome select applications where it is the topology of choice. How do designersget away with building such a convert-Figure 7. Analysis can also be done with PSpice. This figure shows a specific design example for a 15 W converter. Parasitic resistances are included in the PSpice model.Figure 8. This shows the control-to-output transfer function for the Sepic converter. With low values of damping resistors, the converter has four poles, and three right-half-plane zeros. This results in an extreme phase delay of 630 degrees!。
- 1、下载文档前请自行甄别文档内容的完整性,平台不提供额外的编辑、内容补充、找答案等附加服务。
- 2、"仅部分预览"的文档,不可在线预览部分如存在完整性等问题,可反馈申请退款(可完整预览的文档不适用该条件!)。
- 3、如文档侵犯您的权益,请联系客服反馈,我们会尽快为您处理(人工客服工作时间:9:00-18:30)。
Байду номын сангаас
previously determined, through laboratory tests, to be EM1 coupled into the system through the interconnect cabling. The Radar Altimeter system evaluated here consists of a Indicator/Receiver/Transmitter (IRT) and a Remote Indicator (RI) interconnected with a cable harness made up of two shielded twisted pairs, four shielded single wires, and six unshielded wires. The purpose of this paper is to examine the characteristics of the bulk cable current produced by the two test methods to determine the advantages or limitations that one method may have over the other. All tests reported in this paper were performed at a single frequency in the UHF band at which the Radar Altimeter is susceptible. All distances in this report are normalized to the free-space wavelength of the test frequency. All data points presented in this paper represent the average value after five test repetitions.
GENERIC TEST SETUP
Electromagnetic Interference (EMI) caused by radiated signals in the HF, VHF, and UHF bands is often caused by the signals coupling to the system interconnect cables and conducting into an electronic unit to upset the system response. The effectiveness of the shielding of system interconnect cables to prevent EM1 can be evaluated either by exposing the cable to a radiated field from an antenna or by injecting RF signals on the cable bundle using a current injection probe. This paper compares these two test techniques applied to two test cases. The first test case is a simple multiwire cable mounted above a ground plane and terminated at both ends by resistors. This test case serves as a controlled experiment in which various cable shield termination configurations and termination resistance values can be evaluated using bulk cable current injection (BCCI) and radiated fields to induce current on the wiring. The second test case is a helicopter Radar Altimeter system with a known susceptibility to radiated RF signals in the UHF band. The susceptibility is evidenced by variations in the digital altitude. The cause of the ‘Radar Altimeter susceptibility has been
ABSTRACT
Bulk cable current injection of modulated radio frequency (RF) signals will be included in MIL-STD-461D and MIL-STD-462A as conducted susceptibility Test Method CS114. Test Method to evaluate the CS114 is designed susceptibility of electronic equipment to RF signals coupled into the equipment through the cabling. This paper presents an examination of cable current produced by bulk cable current injection and by a radiated field for two test cases; a simple multi-wire cable mounted above a ground plane with each wire terminated with resistors at both ends, and an operational Radar Altimeter system mounted on a mockup of an aircraft instrument panel. For the simple multi-wire test case, the bulk cable current induced on the cable by a current injection probe and by a radiated field is examined for two cable shield termination configurations and two wire termination resistances. The Radar Altimeter, with a known cable-coupled digital altitude susceptibility, is evaluated for several cable shield terminations configurations.
Because of the simplicity, a multiconductor cable mounted over a ground plane and terminated with fixed resistors at both ends was chosen as a general, or generic, case for comparing the BCCI and radiated field methods. This test case is designed to minimize test uncertainty such that insight can be gained into the consequences of variations in the test item. Two identical length multi-wire cables were constructed for the generic tests. The length of the cables was chosen to be the same as the Radar Altimeter interconnect cable. The first cable consists of eight unshielded 20 gauge wires attached to circular military connectors at each end. The second cable consists of eight shielded 22 gauge wires attached to identical circular military connectors at each end. The individual shields of this cable assembly are “daisychained” and terminated to the EM1 backshells on the connectors at each end of the cable. Both cable assemblies were constructed such that a constant cross-section exists along the length of the cable. This is to say that all wires remain parallel in the same relative location in the bundle with no twist along the length of the cable. This was done such that any correlation between variations in individual wire currents and wire location in the bundle could be evaluated. Two identical cable termination boxes were constructed €or the generic tests. These