Reversible plasticity in amorphous materials
严重塑性变形

(011)m
(a)
200nm
(b)
200nm
Strain-induced martensites were formed at the sites of twins intersection. a) bright field and b) dark field imaged using the martensitic reflection. Bright and red arrow indicates the martensite with different sizes. 应变诱导马氏体在孪晶片层间形成
450 µ m deep
500 nm
600 µ m deep
500 nm
500 nm
Cu: X-TEM for the refined layer (36~200 mm deep)
36 µ m deep 80 µ m deep
130 µ m deep
(c)
200 µ m deep
(d)
100 nm
Fe: planar-view TEM images of the top surface layer
nm-sized crystallites with random orientations
200
150
BF image
DF image
Count
D=12 nm (XRD)
100
50
0 5 10 15 20
累积叠轧
Part III
SMAT Surface Mechanical Attrition Treatment
表面机械研磨处理
Nanostructured materials: Bulk vs Surface 纳米结构材料:块体 vs 表面
高分子专业英语讲稿
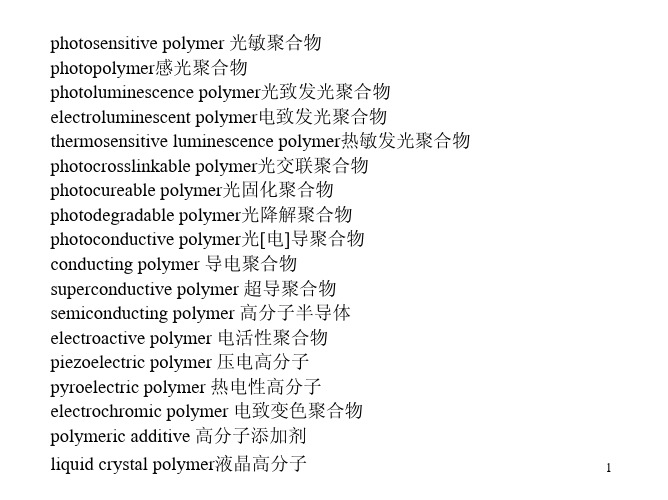
as 引出的宾语补语有如下几种表示形式: (a). 名词 We usually define energy as the ability to do work. (b). 形容词 We often regard gas as compressible. We accept the conclusion as true. (c). 介词短语 We regard the conclusion as of consequence. (具有重要意义的) of consequence: 重要, 有名 (d). 分词或分词短语 (participle 分词) We treat the wire as disconnected. We consider the machine as representing the best one in our factory.
4
As 引出宾语补语、主语补语 (complement 补语) 1. As 引出宾语补语 We regard the sun as the chief source of heat and light. We consider radio waves as radiant (辐射的)energy. 科技英语中,常见如下结构: “动词(或动词性词组)+名词(或代词)+as+宾语补语” (a) to accept …as (b) to define… as (c) to consider…as (d) to refer to…as (e) to treat…as (f) to describe…as (h) to regard…as 把…承认为,承认…是 把…解释为,对…下的定义是 把…认为是 把…指为(叫做) 把…当作;以…来对待 把…描述为 把…看成
8
From the viewpoint of 根据……的观点 From the point of view of From the standpoint of 根据……的观点,站在…的立场上 think of…… as……把……认为是 “主语+被动态谓语+as+-ing”, “-ing”作主语补语 Water can be shown as containing admixtures (混合物). 可以证明水含有杂质 Only those substances which can be considered as being mixtures have a depressed melting point. 那些物质可以被认为是混合物,只有它们才具有较低的熔点。 * -ing 是being 时,being 常可以省略 This may be taken as (being) a result of overheating. More than one type of mistake may be thought of as (being) present.
水滑石复合水泥基材料氯离子吸附能力的研究进展
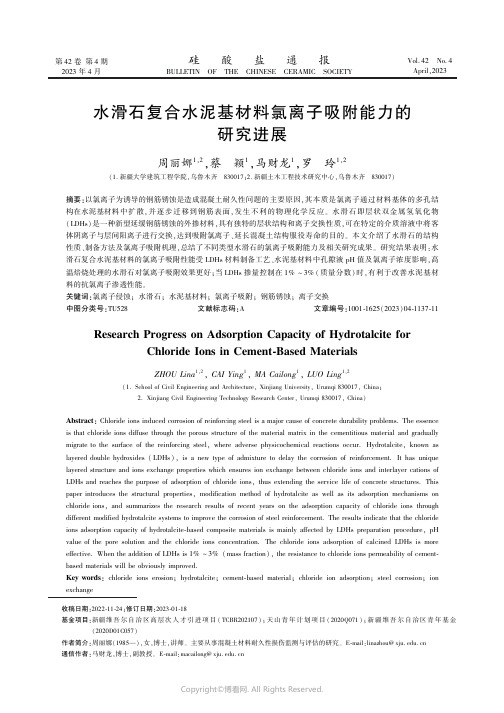
第42卷第4期2023年4月硅㊀酸㊀盐㊀通㊀报BULLETIN OF THE CHINESE CERAMIC SOCIETYVol.42㊀No.4April,2023水滑石复合水泥基材料氯离子吸附能力的研究进展周丽娜1,2,蔡㊀颖1,马财龙1,罗㊀玲1,2(1.新疆大学建筑工程学院,乌鲁木齐㊀830017;2.新疆土木工程技术研究中心,乌鲁木齐㊀830017)摘要:以氯离子为诱导的钢筋锈蚀是造成混凝土耐久性问题的主要原因,其本质是氯离子通过材料基体的多孔结构在水泥基材料中扩散,并逐步迁移到钢筋表面,发生不利的物理化学反应㊂水滑石即层状双金属氢氧化物(LDHs)是一种新型延缓钢筋锈蚀的外掺材料,具有独特的层状结构和离子交换性质,可在特定的介质溶液中将客体阴离子与层间阳离子进行交换,达到吸附氯离子㊁延长混凝土结构服役寿命的目的㊂本文介绍了水滑石的结构性质㊁制备方法及氯离子吸附机理,总结了不同类型水滑石的氯离子吸附能力及相关研究成果㊂研究结果表明:水滑石复合水泥基材料的氯离子吸附性能受LDHs材料制备工艺㊁水泥基材料中孔隙液pH值及氯离子浓度影响,高温焙烧处理的水滑石对氯离子吸附效果更好;当LDHs掺量控制在1%~3%(质量分数)时,有利于改善水泥基材料的抗氯离子渗透性能㊂关键词:氯离子侵蚀;水滑石;水泥基材料;氯离子吸附;钢筋锈蚀;离子交换中图分类号:TU528㊀㊀文献标志码:A㊀㊀文章编号:1001-1625(2023)04-1137-11 Research Progress on Adsorption Capacity of Hydrotalcite forChloride Ions in Cement-Based MaterialsZHOU Lina1,2,CAI Ying1,MA Cailong1,LUO Ling1,2(1.School of Civil Engineering and Architecture,Xinjiang University,Urumqi830017,China;2.Xinjiang Civil Engineering Technology Research Center,Urumqi830017,China)Abstract:Chloride ions induced corrosion of reinforcing steel is a major cause of concrete durability problems.The essenceis that chloride ions diffuse through the porous structure of the material matrix in the cementitious material and gradually migrate to the surface of the reinforcing steel,where adverse physicochemical reactions occur.Hydrotalcite,known as layered double hydroxides(LDHs),is a new type of admixture to delay the corrosion of reinforcement.It has unique layered structure and ions exchange properties which ensures ion exchange between chloride ions and interlayer cations of LDHs and reaches the purpose of adsorption of chloride ions,thus extending the service life of concrete structures.This paper introduces the structural properties,modification method of hydrotalcite as well as its adsorption mechanisms on chloride ions,and summarizes the research results of recent years on the adsorption capacity of chloride ions through different modified hydrotalcite systems to improve the corrosion of steel reinforcement.The results indicate that the chloride ions adsorption capacity of hydrotalcite-based composite materials is mainly affected by LDHs preparation procedure,pH value of the pore solution and the chloride ions concentration.The chloride ions adsorption of calcined LDHs is more effective.When the addition of LDHs is1%~3%(mass fraction),the resistance to chloride ions permeability of cement-based materials will be obviously improved.Key words:chloride ions erosion;hydrotalcite;cement-based material;chloride ion adsorption;steel corrosion;ion exchange㊀收稿日期:2022-11-24;修订日期:2023-01-18基金项目:新疆维吾尔自治区高层次人才引进项目(TCBR202107);天山青年计划项目(2020Q071);新疆维吾尔自治区青年基金(2020D01C057)作者简介:周丽娜(1985 ),女,博士,讲师㊂主要从事混凝土材料耐久性损伤监测与评估的研究㊂E-mail:linazhou@通信作者:马财龙,博士,副教授㊂E-mail:macailong@1138㊀水泥混凝土硅酸盐通报㊀㊀㊀㊀㊀㊀第42卷0㊀引㊀言混凝土是建筑工程中广泛应用的材料之一,其耐久性能备受国内外学者关注㊂因混凝土自身的孔隙结构,外界环境中的氯离子通过孔隙渗入到混凝土内部,当氯离子浓度在钢筋表面达到一定阈值,钝化膜将会发生破坏,诱发钢筋锈蚀[1],严重影响混凝土结构服役寿命[2-5]㊂为了延缓腐蚀的发生,国内外众多学者和工程师致力于钢筋防腐技术的研究,目前相对成熟的保护技术包括阴极保护㊁防腐涂层㊁镀锌钢筋㊁缓蚀剂等[6]㊂上述方法对于延缓钢筋锈蚀均能发挥一定作用,但存在成本高㊁有毒性㊁难降解等问题㊂因此,需要开发一种直接㊁高效且可持续发展的技术㊂近年来,水滑石(layered double hydroxides,LDHs)因其独特的层状结构和离子交换性质,已成为一种新型的外掺材料,应用于混凝土结构中延缓钢筋腐蚀[7-8]㊂2003年Tatematsu 等[9]最早提出类似钙铝类水滑石材料可作为盐类吸附剂用于水泥基材料中,Raki 等[10]进一步证明类水滑石相材料掺入混凝土可控制有机混合物的释放速率㊂基于此,国内外学者从改性的角度出发,探究LDHs 对水泥基材料氯离子吸附性能的影响㊂在钢筋防腐方面,Hong 等[11]和Chen 等[12]通过试验研究表明LDHs 在含氯化物的模拟混凝土孔隙液中表现出良好的耐腐蚀性能,前者设计的MgAl-LDHs 膜用于钢筋基体上,能有效抑制钢筋锈蚀,后者制备的Ca-Al-NO 3LDHs 改性胶凝涂层具有固结氯离子和释放缓释阴离子的双重能力㊂上述研究表明,LDHs 固化氯离子效果显著㊂本文从LDHs 的结构性质和形成机制出发,引入LDHs 的氯离子吸附机理,考虑了LDHs 类型㊁制备方法及水泥基材料孔隙溶液中的pH 值㊁氯离子浓度等主要影响因素,对国内外学者针对不同类型LDHs 复合水泥基材料吸附氯离子能力的研究成果进行归纳梳理,以期为LDHs 在延缓混凝土结构钢筋锈蚀的运用提供理论依据,为延长钢筋混凝土建筑物和构筑物服役寿命提供新思路㊂1㊀水滑石复合水泥基材料1.1㊀水滑石的结构和性质图1㊀LDHs 结构示意图[15]Fig.1㊀Structure schematic diagram of LDHs [15]LDHs 是具有典型层状结构的纳米材料,其化学通式为[M 2+1-x M 3+x (OH)2][A n -]x /n ㊃y H 2O,其中M 2+和M 3+分别表示层板结构中的二价和三价金属阳离子,常见的二价金属阳离子M 2+包括Mg 2+㊁Mn 2+㊁Fe 2+㊁Co 2+等,常见的三价金属阳离子M 3+包括Cr 3+㊁Fe 3+㊁Mn 3+㊁Ga 3+等,A n -为层间的n 价无机(有机)阴离子,如NO -3㊁CO 2-3㊁Cl -㊁OH -㊁SO 2-4等,x 是M 3+/(M 2++M 3+)的摩尔比,一般在0.20~0.33;y 是每个水滑石分子中结晶水的个数[13-14]㊂典型的LDHs 结构[15]如图1所示㊂LDHs 具有离子交换特性,因此也被称为阴离子黏土[16]㊂在钢筋防腐方面,层间阴离子可以与外部环境中存在的侵蚀阴离子置换㊂若预先制备插层阴离子具有缓蚀效果的LDHs,还可在捕捉水泥基材料中游离氯离子的同时,释放抑制腐蚀的阴离子,发挥LDHs 的 双重效应 [17-18]㊂在进行层间交换时,一般需遵循层间阴离子的亲和力顺序:CO 2-3>SO 2-4>OH ->F ->Cl ->Br ->NO -3[19]㊂基于热稳定性和记忆效应,焙烧改性处理后的LDHs 置于特定介质溶液中,可以完成结构重建[20]㊂1.2㊀水滑石的制备水泥熟料中铝酸三钙(C 3A)和铁铝酸四钙(C 4AF)可促进水化产物单硫型水化硫铝酸钙(AFm)的形成,进而完成对氯离子的化学吸附[21-22]㊂AFm 相类属水滑石家族,证实了LDHs 复合水泥基材料使用的可行性[23]㊂水泥中活性MgO 和Al 2O 3的相对含量是形成LDHs 的关键[24],除了天然存在形式,水泥基材料中的LDHs 还可通过外掺的方式获得,根据水泥基材料的性能要求选择合适的掺量㊂外掺LDHs 常用制备工艺包括四种方法:共沉淀法㊁离子交换法㊁水热法和焙烧还原法㊂第4期周丽娜等:水滑石复合水泥基材料氯离子吸附能力的研究进展1139㊀1)共沉淀法共沉淀法为最常用的制备方法,即在一定温度下,将两种金属盐的混合溶液加入碱性溶液中,发生共沉淀以制备LDHs [25-26]㊂朱清等[27]以沉淀剂和温度作为制备MgAl-LDHs 的变量,结果显示,采用NaOH 为沉淀剂㊁晶化温度为70ħ时制备出的MgAl-LDHs 结晶度较好,晶相单一,晶体结构一致㊂Cao 等[28]采用共沉淀法成功制备了CaAl-LDHs,结果表明不同含量的CaAl-LDHs 对硅酸三钙(C 3S)和铝酸四钙(C 4A)的水化速度影响不同,进而使得浆体早强性能不同㊂这一发现为LDHs 在水泥基材料中的实际应用提供理论支持㊂2)离子交换法离子交换法主要基于LDHs 层间离子交换 的特性,根据离子的亲和力大小,进行主客体交换,以得到特定的阴离子插层的LDHs [29]㊂值得注意的是,用于制备盐溶液中的阴离子(即目标阴离子)亲和力应大于原有层间阴离子㊂离子交换法的优点在于反应时间短,是合成非CO 2-3插层LDHs 的常用方法㊂图2为离子交换法示意图㊂图2㊀离子交换法示意图[15]Fig.2㊀Schematic diagram of ion exchange method [15]3)水热法水热法是一种湿化学方法,在密闭压力容器内完成,利用高压㊁高温的水溶液使不溶或难溶的物质通过溶解或反应生成该物质的溶解产物,使其呈过饱和态,进而使得结晶生长[30]㊂水热法的优点是,制备过程在封闭环境中进行,反应产物受到大气环境中CO 2影响较小,产物结晶度好[31]㊂Chen 等[32]和杨成梅等[33]通过水热法,分别合成以硝酸根(NO -3)为插层的LDHs 和Ca-Al-Cl LDHs㊂前者研究了不同LDHs 的氯离子吸附规律,后者研究发现,复配比例下改性的LDHs 结构紧密,提高了水泥注浆早强性能㊂4)焙烧还原法焙烧还原法是根据LDHs 的记忆效应建立的制备方法㊂其基本原理是,通过对LDHs 进行高温煅烧,得到焙烧改性水滑石(calcined layered double hydroxides,CLDHs),将CLDHs 置于特定的介质溶液中完成结构重建[34]㊂焙烧还原法的优点是,在煅烧过程中脱除CO 2-3,提高客体阴离子的插层率㊂图3为CLDHs 层状结构的重建过程㊂图3㊀CLDHs 的结构重建示意图[35]Fig.3㊀Schematic diagram of CLDHs structural reconstruction [35]1140㊀水泥混凝土硅酸盐通报㊀㊀㊀㊀㊀㊀第42卷对比四种常用的水滑石制备方法可知,共沉淀法操作便捷,制得LDHs 结晶度好,其余方法均需要LDHs 作为前驱体进行制备㊂然而,对LDHs 进行改性通常采用焙烧还原法,这归因于其充分利用LDHs 的热稳定性和记忆效应等性质㊂1.3㊀水滑石复合水泥材料吸附机理图4㊀不同处理方法下Mg-Al-CO 3LDHs 的XRD 谱[37]Fig.4㊀XRD patterns of Mg-Al-CO 3LDHs under different treatment methods [37]LDHs 的层状结构和离子交换等特性,使其具有显著的离子吸附性能㊂其中,LDHs 的阴离子吸附能力较CLDHs 弱,可归因于焙烧处理后的LDHs 活性中心增加,对介质溶液中阴离子和水分的吸附能力增强[36],图4为Mg-Al-CO 3LDHs 在不同处理方法下的物相分析,可观察到原状LDHs(即O-LDHs)表现出良好的结晶状态,在经过600ħ的高温焙烧后,LDHs 层状结构发生改变,LDHs 衍射峰由MgO 所取代㊂而经过再水化过程的焙烧水滑石R-LDHs 的衍射峰型和角度与O-LDHs 基本一致[37]㊂离子交换平衡不仅受LDHs 的类型㊁摩尔比和制备方法等因素的影响,还受介质溶液浓度㊁温度和pH值的影响[38-39]㊂目前,众多学者采取吸附等温线确定平衡吸附量㊂最常用的平衡吸附模型分别为Langmiur 和Freundlich 模型,其中,Langmuir 等温线是均匀吸附表面的单分子吸附模型㊂Zuo 等[40]㊁Xu 等[41-42]㊁Chen 等[43]㊁Yoon 等[44]研究均表明Langmiur 吸附等温线模型与试验数据的拟合效果好,可归因于LDHs 的电中性,每个吸附位点只能固定一个氯离子,等温吸附发生在均匀表面㊂研究结果表明,插层为NO -3的LDHs 氯离子吸附量普遍大于插层为NO -2的LDHs 氯离子吸附量,这是由于NO -2插层的LDHs 基底间距小于NO -3插层的LDHs,这使得NO -2插层的LDHs 进行阴离子交换相对困难[41]㊂此外,在同一介质溶液中进行吸附时,共沉淀法吸附效率高于焙烧还原法和水热法㊂对于焙烧还原法,这可能是焙烧过程中温度对结构的破坏引起的㊂水热法吸附量低的原因在于交换过程受产物中的杂质和环境中CO 2的影响㊂当溶液中存在其他竞争性阴离子(如SO 2-4㊁CO 2-3),且浓度高于氯离子浓度时,竞争性阴离子会干扰氯离子吸附,对LDHs 的吸附氯离子性能产生不利影响[42-43]㊂不同类型LDHs 氯离子吸附量见表1㊂表1㊀不同类型LDHs 氯离子吸附量Table 1㊀Chloride ion adsorption capacity of different types of LDHsLDHs type Molar ratio of M 2+to M 3+Synthetic method Solution composition pH value Experimental condition Adsorption capacity /(mmol㊃g -1)Ref.MgAl-NO 21ʒ1Coprecipitation NaCl 7Room temperature 3.61[40]MgAl-NO 23ʒ1Calcination-rehydration NaCl 7Room temperature 1.6[40]MgAl-NO 21ʒ1Hydrothermal NaCl 7Room temperature 2.55[40]MgAl-NO 22ʒ1Ion exchange Ca(OH)2+NaCl 12.625ħN 2atmosphere 2.51[41]MgAl-NO 32ʒ1Coprecipitation Ca(OH)2+NaCl 12.625ħN 2atmosphere 3.61[41]MgAl-NO 32ʒ1Coprecipitation NaCl +NaOH 1325ħN 2atmosphere 2.74[42]MgAl-NO 32ʒ1Coprecipitation NaCl +NaOH +Na 2SO 41325ħN 2atmosphere 0.65[42]CaAl-NO 32ʒ1Coprecipitation NaCl 7Room temperature 25ħ 3.38[43]CaAl-NO 32ʒ1Coprecipitation Cement mortar 4.5[43]MgAl-pAB 1ʒ1Calcination-rehydration Cement paste Room temperature 20ħ 4.31[44]2㊀不同类型水滑石的氯离子吸附性能2.1㊀焙烧改性水滑石基于LDHs 的阴离子交换㊁记忆效应以及结构重建等特性,可以推断出,与LDHs 相比,经过高温焙烧后的CLDHs 的吸附氯离子性能更优㊂这归因于CLDHs 层间出现较多的活性中心,可吸附较多的氯离子,从而第4期周丽娜等:水滑石复合水泥基材料氯离子吸附能力的研究进展1141㊀完成层状结构的重建[44]㊂图5(a)和(b)分别为焙烧改性原状水滑石CLDHs 和在水泥基材料中完成结构重建的LDHs 的SEM 照片㊂从微观形貌上看,前者样品颗粒边缘尖锐锋利,而后者在水泥基质中完成离子交换后,具有明显的片状结构且层层堆叠在一起㊂图5㊀不同环境中水滑石在水泥基质中的SEM 照片[44]Fig.5㊀SEM images of LDHs in different environment conditions in cement paste[44]图6㊀钢筋在模拟孔隙液及CLDHs 处理前后的Nyquist 图[46]Fig.6㊀Nyquist plots for rebar in simulated pore solution with /without CLDHs [46]混凝土中掺入的CLDHs 吸附氯离子,可提高钢筋表面的氯离子阈值,从而延缓钢筋锈蚀开始的时间㊂图6为钢筋在模拟孔隙液(simulated pore solution,SPS)及CLDHs 处理前后的Nyquist 图,唐聿明等[45]和牛乐[46]发现经CLDHs 处理的孔隙液,电容弧直径下降明显,这表明处理后的孔隙液对钢筋的侵蚀作用减小㊂水滑石在水泥基材料体系与模拟孔溶液中发挥的固氯作用存在不一致的结论㊂CLDHs 对氯离子的吸附是利用其结构记忆效应的化学吸附,胡静等[47]和张琳[48]针对MgAl-CO 3CLDHs,在模拟孔隙液中通过吸附热力学对其吸附氯离子机理进行基础研究㊂在此基础上,张琳进一步探究了CLDHs 在水泥净浆中的固氯能力和机理,结果表明,与对照组相比,掺CLDHs 的试验组固氯能力更好,值得关注的是,CLDHs 固氯能力随养护龄期的增长呈增长趋势,且固氯能力与氯离子浓度呈正相关㊂混凝土中的氯离子可分为结合氯离子和自由氯离子,一般认为自由氯离子会破坏钢筋界面的钝化膜[49]㊂王佩[50]研究表明,掺入的CLDHs 填充水泥基材料的孔隙,其密实度提升,抗冻能力增强,固氯能力随LDHs 掺量的增加而提高㊂基于LDHs 的离子交换功能,段平等[51]㊁Ma 等[52]和Shui 等[37]评估了不同处理方式得到图7㊀掺LDHs 混凝土氯离子扩散系数[58-60]Fig.7㊀Chloride diffusion coefficient of concrete mixed with LDHs [58-60]的CLDHs 混凝土的抗碳化性能㊂上述研究结果表明,CLDHs 的吸附氯离子性能优于LDHs㊂Liu 等[53]验证了这一结论㊂作为替代水泥的外掺材料,LDHs 的掺量须结合水泥基材料拌合物的工作性能进行调节和控制㊂冯跃等[54]㊁Wang 等[55]和宋学锋等[56]分别针对水滑石复合水泥基材料的氯离子侵蚀和碳化问题进行了相应研究㊂在不影响砂浆整体强度的条件下,CLDHs 的最佳掺量控制在胶凝材料质量的1%~3%㊂过量掺入LDHs 会影响水泥水化进程,对水泥基材料的孔隙结构和强度造成不利影响,从而影响水泥基材料抗氯离子渗透的能力[57]㊂图7为学者们采用快速氯离子迁移1142㊀水泥混凝土硅酸盐通报㊀㊀㊀㊀㊀㊀第42卷法表征不同LDHs 掺量下的水泥基材料的氯离子吸附能力[58-60]㊂由图7可知,混凝土中氯离子迁移系数D RCM 随LDHs 的掺量的增加呈先减小后增大的趋势㊂氯离子迁移系数减小的原因在于LDHs 的掺入降低混凝土基体孔隙率,提高其抗氯离子渗透能力,而LDHs 比表面积较大,拌和时吸附水泥基体中的水分,过量掺入使水化反应不充分,Ca(OH)2的生成量减少,对混凝土的孔隙结构产生不利影响,进而导致抗氯离子渗透能力降低㊂图8为段平[36]㊁马军涛[58]㊁Ma 等[61]㊁Wang 等[62]采用NT-Build 443法测定氯盐环境下不同LDHs 掺量混凝土氯离子浓度分布情况㊂由图8可知,复掺LDHs 混凝土内部不同深度氯离子浓度均呈递减趋势,且较对照组浓度低㊂呈这种变化趋势的原因:一方面是LDHs 的掺入会减少混凝土内部的毛细孔数量,改善混凝土的微观结构,阻碍氯离子通过孔隙向混凝土内部扩散的进程;另一方面是LDHs 会吸附部分氯离子,延缓了氯离子向内部渗透的进程㊂图8㊀氯盐环境下掺LDHs 试块的氯离子分布曲线[36,58,61-62]Fig.8㊀Chloride ion distribution curves of specimens mixed with LDHs in chloride salt environment [36,58,61-62]2.2㊀阻锈阴离子插层水滑石阻锈阴离子吸附效能取决于其自身同外界氯离子之间的亲和力顺序[63-64],即LDHs 层间阴离子亲和力越低,越容易被外界氯离子所代替,其固氯能力就越强,对钢筋防腐性能和混凝土耐久性能提升效果就越显著㊂因此,国内外学者通过不同方法合成具有阻锈能力或缓蚀能力的LDHs,探讨其吸附氯离子的能力㊂其中,可发现插层多为NO -3或NO -2㊂从XRD 的角度对插层分别为NO -3和NO -2的LDHs 的氯离子吸附能力进行研究,如图9所示㊂由图可知,二者在氯离子吸附前后,均存在明显的LDHs 衍射特征峰,这表明样品结晶良好㊂从图9(a)可以看出,在氯离子吸附前后,LDHs-NO -3基底间距从0.8717nm 降低至0.7890nm,这是由于氯离子直径小于NO -3的直径;从图9(b)可以看出,在氯离子吸附前后,LDHs-NO -2基底间距从0.7702nm 略升至0.7771nm,这是由于氯离子与NO -2在直径上较相近㊂因此,在进行离子交换时,LDHs-NO -3较LDHs-NO -2相对容易,且前者的氯离子吸附量大于后者㊂Yang 等[60]㊁Cao 等[65]㊁Chen 等[66]和Yang 等[67]制备了插层阴离子具有缓蚀效果的LDHs,均可在钢筋混凝土中发挥双重效应,捕捉水泥基材料中的游离氯离子,同时向水泥基材料中释放阻锈阴离子,有效控制混凝土中钢筋的锈蚀,但是须考虑LDHs 的掺量,Yang 等[67]指出过量使用LDHs 会增加混凝土的孔隙率,为氯离子的传输提供途径㊂ZnAl-NO 2LDHs 缓蚀性能优异,其对钢筋的缓蚀效率受pH 值的影响较显著㊂Gomes 等[68]认为孔隙液在中性pH 值条件下,ZnAl-NO 2LDHs 具有良好的氯捕集能力,但随着pH 值的增加,氯捕集能力降低,这是由于孔隙液中的OH -会占据LDHs 结构中的结合位点㊂Cao 等[69]认为孔隙液在碱性pH 值条件下,插层离子的释放不仅受OH -对LDHs 的亲和力的影响,也受LDHs 部分溶解的影响,但目前,关于LDHs 在碱性溶液中发生部分溶解对缓蚀率的影响还未开展系统研究㊂此外,田玉琬等[70]认为可根据孔隙液中氯离子浓度智能释放NO -2,高碱环境下的释放效果主要受氯离子控制㊂第4期周丽娜等:水滑石复合水泥基材料氯离子吸附能力的研究进展1143㊀图9㊀氯离子吸附前后LDHs 的XRD 谱[41]Fig.9㊀XRD patterns of LDHs before and after chloride adsorption [41]2.3㊀复合防御体系水滑石LDHs 在水泥基材料中的形成机制包括两种:受掺合料中镁铝氧化物相对含量影响的MgAl-LDHs 和具有水化产物AFm 相结构的CaAl-LDHs [71]㊂Kayali 等[72]通过快速氯离子渗透法㊁XRD 等测试方法对掺有矿渣的混凝土试样进行研究,发现LDHs 作为一种重要的水化产物在矿渣混凝土中生成,并显示出优越的固氯能力,以延缓混凝土结构中的钢筋锈蚀㊂LDHs 吸附侵蚀阴离子,复合掺入矿物掺合料,发挥二者的叠加效应,使胶凝材料的颗粒级配更为合理,在微集料效应作用下,完善混凝土内部微观结构,显著提升混凝土综合性能㊂部分学者通过矿物掺合料与LDHs 复掺提高混凝土的抗氯离子侵蚀能力及耐久性,并取得了一定的成果㊂图10㊀复合体系在氯盐环境下的氯离子分布曲线[58,73]Fig.10㊀Chloride ion distribution curves of composite system in chloride salt environment [58,73]混凝土抗氯离子侵蚀性能测试可按照NTBuild-443非稳态自然扩散法进行,马军涛[58]和陈宇轩等[73]采用此法研究了LDHs 与偏高岭土(metakaolin,MK)为主的矿物掺合料复合使用,对混凝土抗氯离子渗透性能的改善情况,并得出氯离子扩散浓度同扩散距离二者之间的规律㊂图10为二者采用NTBuild-443的数据对比,由图可见,相较于对照组,复合体系下的LDHs提升了混凝土抗氯离子侵蚀的能力㊂LDHs 材料主要通过煅烧处理后的结构重建过程和离子交换实现对氯离子的吸附,掺加改性剂的混凝土可进一步改善其抗氯离子渗透性,提高混凝土的抗氯离子侵蚀能力㊂陈国玮等[74]发现LDHs 材料可有效吸附外界侵入的SO 2-4,而MK 在混凝土中的火山灰特性可促进水泥的水化过程,改善混凝土内部的显微结构㊂3㊀结语与展望本文从LDHs 结构特性角度出发,综述了不同类型LDHs 体系对氯离子吸附能力的研究现状,对于探明复掺LDHs 水泥基材料体系的固氯能力和延缓钢筋锈蚀的机理提供了理论基础,得到的主要结论如下:1)LDHs 的氯离子吸附能力受制备工艺的影响,通过吸附等温模型评估了不同制备方法所得的LDHs 氯离子吸附量㊂其中,Langmiur 模型对试验数据的拟合效果最好,且共沉淀法制备的LDHs 氯离子吸附量优于水热法和焙烧还原法㊂2)水泥基材料孔隙液的pH 值和氯离子浓度对LDHs 的氯离子吸附性能有一定的影响㊂当孔隙液在高碱环境下或存在其他竞争性离子,且浓度高于氯离子浓度时,竞争性离子会率先占据水滑石层间的结合位1144㊀水泥混凝土硅酸盐通报㊀㊀㊀㊀㊀㊀第42卷点,影响LDHs对孔隙液中氯离子的捕捉㊂3)LDHs的氯离子吸附能力主要取决于其独特的层状结构和离子交换性质㊂CLDHs利用记忆效应,在结构重建的过程中完成对水泥基材料孔隙液中氯离子吸附,阻锈阴离子插层LDHs利用层间离子交换功能,在捕捉氯离子的同时,释放具有缓蚀效果的阴离子,高温焙烧处理的水滑石对氯离子吸附效果更好;复合防御体系LDHs发挥叠加效应,在LDHs吸附机理基础上,从改善孔隙结构的角度改善氯离子侵蚀㊂然而,由于实际试验的局限,对不同种类的LDHs的吸附效果难以进行精确的量化分析㊂目前,关于LDHs在碱性孔隙液中发生部分溶解对缓蚀离子的释放速率影响,以及不同矿物掺合料与LDHs复合对水泥基材料的氯离子吸附效应和机理还需进一步系统研究㊂参考文献[1]㊀杨长辉,晏㊀宇,欧忠文.偏高岭土水泥净浆结合氯离子性能的研究[J].混凝土,2010(10):1-3+7.YANG C H,YAN Y,OU Z W.Capability of cement paste binding chloride ions with metakaolin as admixture[J].Concrete,2010(10):1-3+ 7(in Chinese).[2]㊀TANG S W,YAO Y,ANDRADE C,et al.Recent durability studies on concrete structure[J].Cement and Concrete Research,2015,78:143-154.[3]㊀OTIENO M,BEUSHAUSEN H,ALEXANDER M.Chloride-induced corrosion of steel in cracked concrete-part I:experimental studies underaccelerated and natural marine environments[J].Cement and Concrete Research,2016,79:373-385.[4]㊀XIONG C S,LI W H,JIN Z Q,et al.Preparation of phytic acid conversion coating and corrosion protection performances for steel in chlorinatedsimulated concrete pore solution[J].Corrosion Science,2018,139:275-288.[5]㊀JIN Z Q,CHANG H L,DU F Y,et al.Influence of SAP on the chloride penetration and corrosion behavior of steel bar in concrete[J].Corrosion Science,2020,171:108714.[6]㊀CHEN C,JIANG L H,GUO M Z,et al.Effect of sulfate ions on corrosion of reinforced steel treated by DNA corrosion inhibitor in simulatedconcrete pore solution[J].Construction and Building Materials,2019,228:116752.[7]㊀GOMES C,MIR Z M,BASTOS A C,et al.Effect of layered double hydroxides on the performance and service life of reinforced concrete[C]//Conference on Durable Concrete for Infrastructure under Severe Conditions-Smart Admixtures,Self-Responsiveness and Nanoadditions,Ghent, Belgium,September,2019,10-11.[8]㊀MIR Z M,BASTOS A,HÖCHE D,et al.Recent advances on the application of layered double hydroxides in concrete-a review[J].Materials(Basel,Switzerland),2020,13(6):1426.[9]㊀TATEMATSU H,SASAKI T.Repair materials system for chloride-induced corrosion of reinforcing bars[J].Cement and Concrete Composites,2003,25(1):123-129.[10]㊀RAKI L,BEAUDOIN J J,MITCHELL yered double hydroxide-like materials:nanocomposites for use in concrete[J].Cement and ConcreteResearch,2004,34(9):1717-1724.[11]㊀HONG S X,QIN S F,LIU Z M,et al.Enhanced corrosion resistance and applicability of Mg/Al-CO2-3layered double hydroxide film on Q235steel substrate[J].Construction and Building Materials,2021,276:122259.[12]㊀CHEN M Z,WEI Y N,ZHENG H B,et al.Ca-LDH-modified cementitious coating to enhance corrosion resistance of steel bars[J].Journal ofBuilding Engineering,2022,51:104301.[13]㊀OESTREICHER V,JOBBÁGY M,REGAZZONI A E.Halide exchange on Mg(II)-Al(III)layered double hydroxides:exploring affinities andelectrostatic predictive models[J].Langmuir:the ACS Journal of Surfaces and Colloids,2014,30(28):8408-8415.[14]㊀SJÅSTAD A O,ANDERSEN N H,VAJEESTON P,et al.On the thermal stability and structures of layered double hydroxidesMg1-x Al x(OH)2(NO3)x㊃m H2O(0.18ɤxɤ0.38)[J].European Journal of Inorganic Chemistry,2015,2015(10):1775-1788. [15]㊀YANG Z X,FISCHER H,CEREZO J,et al.Aminobenzoate modified MgAl hydrotalcites as a novel smart additive of reinforced concrete foranticorrosion applications[J].Construction and Building Materials,2013,47:1436-1443.[16]㊀孙㊀远.不同形貌水滑石及其焙烧产物的离子吸附性能研究[D].哈尔滨:哈尔滨工业大学,2019.SUN Y.Research on ion adsorption properties of hydrotalcite with different morphologies and its calcined products[D].Harbin:Harbin Institute of Technology,2019(in Chinese).[17]㊀BALONIS M,GLASSER F P.Calcium nitrite corrosion inhibitor in Portland cement:influence of nitrite on chloride binding and mineralogy[J].Journal of the American Ceramic Society,2011,94(7):2230-2241.[18]㊀YE H L.Autogenous formation and smart behaviors of nitrite-and nitrate-intercalated layered double hydroxides(LDHs)in Portland cement-metakaolin-dolomite blends[J].Cement and Concrete Research,2021,139:106267.[19]㊀COSTA D G,ROCHA A B,SOUZA W F,et parative structural,thermodynamic and electronic analyses of Zn-Al-A n-hydrotalcite-like㊀第4期周丽娜等:水滑石复合水泥基材料氯离子吸附能力的研究进展1145 compounds(A n-=Cl-,F-,Br-,OH-,CO2-3or NO-3):an ab initio study[J].Applied Clay Science,2012,56:16-22.[20]㊀NEVILLE A.Chloride attack of reinforced concrete:an overview[J].Materials and Structures,1995,28(2):63-70.[21]㊀JUSTNE H.A review of chloride binding in cementitious systems[J].Nordic Concrete Research,1998,21:48-63.[22]㊀PROVIS J L,BERNAL S A.Geopolymers and related alkali-activated materials[J].Annual Review of Materials Research,2014,44:299-327.[23]㊀BERNAL S A,SAN NICOLAS R,MYERS R J,et al.MgO content of slag controls phase evolution and structural changes induced by acceleratedcarbonation in alkali-activated binders[J].Cement and Concrete Research,2014,57:33-43.[24]㊀许㊀闯,张祖华,陈慕翀,等.层状双金属氢氧化物在水泥混凝土中的形成㊁作用机制及应用[J].材料导报,2022,36(11):99-105.XU C,ZHANG Z H,CHEN M C,et al.Formation,interaction mechanisms and application of layered double hydroxides in cement concrete[J].Materials Reports,2022,36(11):99-105(in Chinese).[25]㊀VACCARI yered double hydroxides:present and future[J].Applied Clay Science,2002,22(1/2):75-76.[26]㊀DUAN X,EVANS D yered double hydroxides:application of layered double hydroxides[J].Structure and Bonding,2006,119:193-223.[27]㊀朱㊀清,李成胜,周建成.镁铝水滑石的共沉淀法制备及表征[J].化工时刊,2017,31(4):1-3+17.ZHU Q,LI C S,ZHOU J C.The Co-precipitation preparation and properties of inorganic hydrotalcite[J].Chemical Industry Times,2017,31(4):1-3+17(in Chinese).[28]㊀CAO L,GUO J T,TIAN J H,et al.Preparation of Ca/Al-layered double hydroxide and the influence of their structure on early strength ofcement[J].Construction and Building Materials,2018,184:203-214.[29]㊀LIU Z P,MA R Z,OSADA M,et al.Synthesis,anion exchange,and delamination of Co-Al layered double hydroxide:assembly of the exfoliatednanosheet/polyanion composite films and magneto-optical studies[J].Journal of the American Chemical Society,2006,128(14):4872-4880.[30]㊀张骄佼,田森林,周㊀键.镁铝水滑石的合成与应用研究进展[J].材料导报,2013,27(7):144-148.ZHANG J J,TIAN S L,ZHOU J.Study on synthesis and application of Mg-Al hydrotalcite[J].Materials Review,2013,27(7):144-148(in Chinese).[31]㊀吴㊀波.阻锈阴离子插层改性水滑石的制备及其在水泥砂浆中的应用[D].深圳:深圳大学,2019.WU B.Preparation of inhibitor ions intercalation modified hydrotalcite and its application in cement mortar[D].Shenzhen:Shenzhen University, 2019(in Chinese).[32]㊀CHEN M Z,WU F,YU L W,et al.Chloride binding capacity of LDHs with various divalent cations and divalent to trivalent cation ratios indifferent solutions[J].CrystEngComm,2019,21(44):6790-6800.[33]㊀杨成梅,谢㊀竺.水滑石/粉煤灰对注浆早强性能的影响研究[J].化学与粘合,2021,43(1):48-51.YANG C M,XIE Z.Research on the effect of hydrotalcite/fly ash on the early strength of cement grouting[J].Chemistry and Adhesion,2021, 43(1):48-51(in Chinese).[34]㊀VON HOESSLE F,PLANK J,LEROUX F.Intercalation of sulfonated melamine formaldehyde polycondensates into a hydrocalumite LDHstructure[J].Journal of Physics and Chemistry of Solids,2015,80:112-117.[35]㊀YANG Z,FISCHER H,CEREZO J,et al.Modified hydrotalcites for improved corrosion protection of reinforcing steel in concrete-preparation,characterization,and assessment in alkaline chloride solution[J].Materials and Corrosion,2016,67(7):721-738.[36]㊀段㊀平.层状双氢氧化物改善混凝土耐久性能的机理及其应用研究[D].武汉:武汉理工大学,2014.DUAN P.Research on modification mechanism and the application of layered double hydroxides for durability of concrete[D].Wuhan:Wuhan University of Technology,2014(in Chinese).[37]㊀SHUI Z H,YU R,CHEN Y X,et al.Improvement of concrete carbonation resistance based on a structure modified layered double hydroxides(LDHs):experiments and mechanism analysis[J].Construction and Building Materials,2018,176:228-240.[38]㊀HANG T T X,TRUC T A,DUONG N T,et yered double hydroxides as containers of inhibitors in organic coatings for corrosion protectionof carbon steel[J].Progress in Organic Coatings,2012,74(2):343-348.[39]㊀NAKAYAMA H,WADA N,TSUHAKO M.Intercalation of amino acids and peptides into Mg-Al layered double hydroxide by reconstructionmethod[J].International Journal of Pharmaceutics,2004,269(2):469-478.[40]㊀ZUO J D,WU B,LUO C Y,et al.Preparation of MgAl layered double hydroxides intercalated with nitrite ions and corrosion protection of steelbars in simulated carbonated concrete pore solution[J].Corrosion Science,2019,152:120-129.[41]㊀XU J X,SONG Y B,ZHAO Y H,et al.Chloride removal and corrosion inhibitions of nitrate,nitrite-intercalated MgAl layered double hydroxideson steel in saturated calcium hydroxide solution[J].Applied Clay Science,2018,163:129-136.[42]㊀XU J X,TAN Q P,MEI Y J.Corrosion protection of steel by Mg-Al layered double hydroxides in simulated concrete pore solution:effect ofSO2-4[J].Corrosion Science,2020,163:108223.[43]㊀CHEN Y X,SHUI Z H,CHEN W,et al.Chloride binding of synthetic Ca-Al-NO3LDHs in hardened cement paste[J].Construction andBuilding Materials,2015,93:1051-1058.[44]㊀YOON S,MOON J,BAE S,et al.Chloride adsorption by calcined layered double hydroxides in hardened Portland cement paste[J].MaterialsChemistry and Physics,2014,145(3):376-386.。
高分子材料专业英语单词及短语

permeability渗透性
in a stepwise fashion逐步的已逐步的方式
statistical统计的
glycerol甘油;
hydroxyl羟基
segment链段
gauche isomerism旁氏异构
in conclusion总之
semicrystalline半晶
sedimentation沉降(法)
folded-chain lamella theory折叠链片晶理论
polydispersity多分散性
viscosity average molecular weight黏均分子量
depending on根据
miscible可混溶的
dibasic二元的
attraction引力,吸引;
diol二元醇
categoty种类,类型;
titanium tetrachloride四氯化钛
tertiary三元的
isotactic无规立构的;
degenerate退化,变性,变质
cause ..to..使...做...
reflux回流
urea-formaldehyde resin脲醛树脂
powdery粉状的
isotropic各向同性的
swollen溶胀的
orient定向,取向;
contaminant污物
mechanism机理
molecular weight distribution分子量分布
relaxation松弛
arrangement排布,排列
step-growth polymerization逐步聚合
precipitation沉淀
复合材料多尺度模型 英语
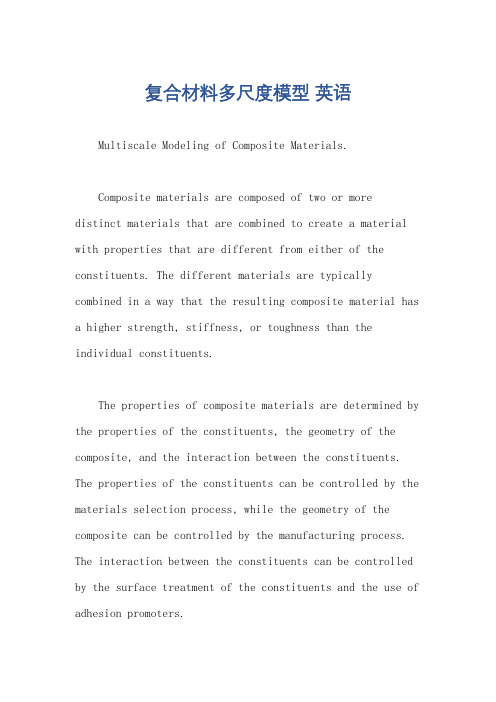
复合材料多尺度模型英语Multiscale Modeling of Composite Materials.Composite materials are composed of two or moredistinct materials that are combined to create a material with properties that are different from either of the constituents. The different materials are typically combined in a way that the resulting composite material has a higher strength, stiffness, or toughness than the individual constituents.The properties of composite materials are determined by the properties of the constituents, the geometry of the composite, and the interaction between the constituents. The properties of the constituents can be controlled by the materials selection process, while the geometry of the composite can be controlled by the manufacturing process. The interaction between the constituents can be controlled by the surface treatment of the constituents and the use of adhesion promoters.The analysis of composite materials is a complex problem due to the multiscale nature of the material. The constituents of a composite material can have different length scales, ranging from the atomic scale to the macroscopic scale. The analysis of a composite material must take into account all of the length scales in order to accurately predict the properties of the material.Multiscale modeling is a technique that is used to analyze composite materials. Multiscale modeling involves dividing the composite material into a series of smaller subvolumes, each of which is analyzed at a different length scale. The results of the analysis of the subvolumes are then combined to create a model of the entire composite material.The use of multiscale modeling allows for the accurate analysis of composite materials, despite the challenges posed by the multiscale nature of the material. Multiscale modeling can be used to predict the mechanical, thermal, and electrical properties of composite materials.Multiscale modeling can also be used to optimize the design of composite materials for specific applications.Benefits of Multiscale Modeling.Multiscale modeling offers a number of benefits over traditional modeling techniques. These benefits include:Accuracy: Multiscale modeling can provide more accurate predictions of the properties of composite materials than traditional modeling techniques. This is because multiscale modeling takes into account thedifferent length scales of the constituents of the composite material.Efficiency: Multiscale modeling can be more efficient than traditional modeling techniques. This is because multiscale modeling can be used to analyze only the relevant length scales of the composite material.Versatility: Multiscale modeling can be used to analyze a wide variety of composite materials. This isbecause multiscale modeling is not limited to a particular length scale or type of composite material.Applications of Multiscale Modeling.Multiscale modeling has been used to analyze a wide variety of composite materials, including:Polymer composites.Metal matrix composites.Ceramic matrix composites.Carbon-fiber-reinforced composites.Glass-fiber-reinforced composites.Multiscale modeling has been used to predict the mechanical, thermal, and electrical properties of composite materials. Multiscale modeling has also been used to optimize the design of composite materials for specificapplications.Challenges of Multiscale Modeling.Despite the benefits of multiscale modeling, there are still a number of challenges associated with this technique. These challenges include:Computational cost: Multiscale modeling can be computationally expensive. This is because multiscale modeling requires the analysis of a large number of subvolumes.Model complexity: Multiscale models can be complex. This is because multiscale models must take into accountthe different length scales of the constituents of the composite material.Data requirements: Multiscale modeling requires alarge amount of data. This data includes the material properties of the constituents of the composite materialand the geometry of the composite material.The challenges of multiscale modeling are being addressed by researchers around the world. The development of new computational techniques and modeling algorithms is helping to reduce the computational cost of multiscale modeling. The development of new modeling techniques is also helping to reduce the complexity of multiscale models. The development of new data acquisition techniques is helping to reduce the data requirements of multiscale modeling.Future of Multiscale Modeling.Multiscale modeling is a powerful tool for the analysis and design of composite materials. Multiscale modeling is still in its early stages of development, but this technique has the potential to revolutionize the way that composite materials are designed and used.。
高分子材料英文论文翻译
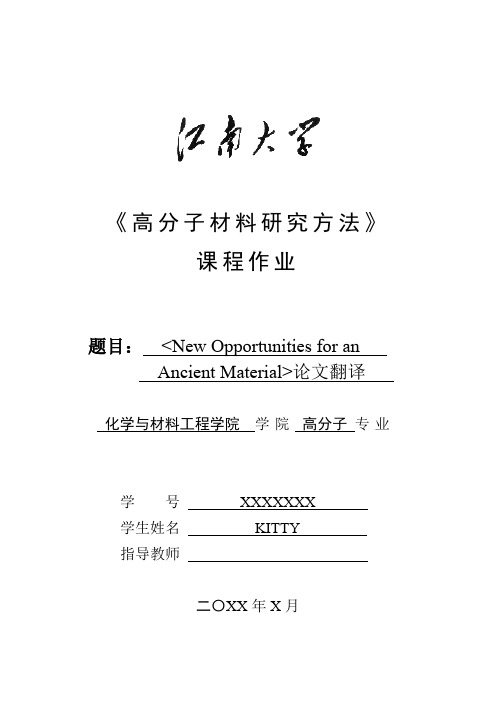
《高分子材料研究方法》课程作业题目:<New Opportunities for anAncientMaterial>论文翻译化学与材料工程学院学院高分子专业学号XXXXXXX学生姓名KITTY指导教师二〇XX年X月<New Opportunities for an Ancient Material>论文翻译Science 30 July 2010:V ol. 329 no. 5991 pp. 528-531DOI: 10.1126/science.1188936New Opportunities for an Ancient MaterialFiorenzo G. Omenetto, David L. Kaplan*Department of Biomedical Engineering, Tufts University, Medford, MA 02155, USA.E-mail: david.kaplan{at}一种古老材料的新机遇Fiorenzo G. Omenetto, David L. Kaplan*美国,马萨诸塞州(邮编02155),梅德福,塔夫茨大学,生物医学工程系邮箱:david.kaplan{at}ABSTRACTSpiders and silkworms generate silk protein fibers that embody strength and beauty. Orb webs are fascinating feats of bioengineering in nature, displaying magnificent architectures while providing essential survival utility for spiders. The unusual combination of high strength and extensibility is a characteristic unavailable to date in synthetic materials yet is attained in nature with a relatively simple protein processed from water. This biological template suggests new directions to emulate in the pursuit of new high-performance, multifunctional materials generated with a green chemistry and processing approach. These bio-inspired and high-technology materials can lead to multifunctional material platforms that integrate with living systems for medical materials and a host of other applications.摘要蜘蛛或桑蚕生成的丝蛋白纤维,是力与美的结合体。
高分子化学专业术语中英文对照

高分子化学专业术语中英文对照中英文对照加聚反应Addition Polymerization加聚物Addition Polymer粘结剂Adhesive老化Ageing交替共聚物Alternating Copolymer交替共聚Alternating Copolymerization无定型态Amorphous State阴离子聚合Anionic Polymerization无规立构Atactic无规立构聚合物Atactic Polymer原子转移自由基聚合Auto Transfer Radical Polymerization, ATRP 自动加速Auto-acceleration平均官能度Average Functionality恒比共聚Azeotropic Copolymerization恒比点Azeotropic Point支化型聚合物Branched Polymer共混改性Blending Modificatioon嵌段共聚物Block Copolymer本体聚合Bulk or Mass Polymerization笼蔽效应Cage Effect碳链聚合物Carbon Chain Polymer阳离子聚合Cationic Polymerization扩链Chain Extension链节Chain Element链引发Chain Initiation连锁聚合Chain Polymerization链增长Chain Propagation链终止Chain Termination链转移Chain Transfer链转移剂Chain Transfer Agent手性中心Chiral Center顺丁橡胶cis-1,4-Polybutadiene rubber,BR涂料Coatings共缩聚Co-condensation Polymerization梳形聚合物Comb-shaped Polymer络合聚合Complexing Polymerization缩聚反应Condensation Polymerization构型异构Configurational Isomerism构象异构Conformational Isomerism转化率Conversion配位聚合Coordination Polymerization共聚物Copolymer共聚合Copolymerization共聚组成Copolymer Composition偶合终止Coupling Termination反离子Counterion临界胶束浓度Critical Micelle Concentration, CMC 交联Crosslinking结晶态Crystalline Morphology降解Degradation聚合度Degree of Polymerization, DP树枝状聚合物Dendritic Polymer解聚Depolymerization分散剂Dispersant歧化终止Disproportionation Termination元素有机高分子Elementary Organic Polymer乳化作用Emulsification乳化剂Emulsifier乳液聚合Emulsion Polymerization乳胶粒Emulsion Particle对映体异构Enantiomer Isomerism环氧树脂Epoxy Resin端基End Group平衡缩聚Equilibrium Polycondensation过量分率Excessive Ratio反应程度Extent of Reaction纤维Fiber功能材料Functional Material功能高分子Functional Polymer官能度Functionality, f几何异构Geometrical Isomerism凝胶点Gel point, Pc凝胶效应Gel Effect凝胶化Gelation玻璃化温度Glass Transition Temperature, Tg 接枝聚合Graft Copolymerization接枝共聚物Graft Copolymer半衰期Half-life杂链聚合物Hetero-chain Polymer高密度聚乙烯High Density Polyethylene HDPE 高抗冲聚苯乙烯High Impact Polystyrene, HIPS 均相成核Homogenous Nucleation均聚物Homopolymer均聚合Homopolymerization均缩聚Homopolycondensation理想共聚Ideal Copolymerization理想恒比共聚Ideal Azeotropic Copolymerization 诱导期Induction Period诱导分解Induced Decomposition阻聚剂Inhibitor阻聚作用Inhibition引发剂Initiator引发效率Initiator Efficiency无机高分子Inorganic Polymer插入聚合Insertion Polymerization原位复合In-situ Compounding界面缩聚Interfacial Polycondensation互穿网络Interpenetrating Polymer Network 离子聚合Ionic Polymerization离子对Ion-pair异构化聚合Isomerization Polymerization等规立构Isotactic等规度Isotacticity动力学链长Kinetic Chain Length梯形聚合物Ladder Polymer自由基寿命Life-time of Free Radical线型缩聚Linear Polycondensation线型聚合物Linear Polymer活性聚合Living Polymerization“活性”/可控自由基聚合“Living”/Controlled Polymerization 低密度聚乙烯Low Density Polyethylene LDPE 高分子(大分子)Macromolecule主链Main Chain熔融缩聚Melt Polycondensation茂金属引发剂Metallocene Initiator胶束成核Micelle Nucleation混缩聚Mixing Polycondensation摩尔系数Molar Coefficient单体Monomer单体单元Monomer Unit单体液滴Monomer Droplet天然高分子Natural Polymer邻位基团效应Neighboring Group Effect交联型聚合物Network Structure Polymer非理想共聚Non-ideal Copolymerization非理想恒比共聚Non-ideal Azeotropic Copolymerization 线型酚醛树脂Novolacs尼龙Nylon尼龙-6 Nylon-6, PA-6尼龙-66 Nylon-66, PA-66光学异构Optical Isomerism齐聚物(低聚物)Oligomer氧化偶合反应Oxidative Coupling Polymerization前末端效应Penultimate Effect光引发Photo initiation塑料Plastics聚酰胺Polyamide, PA聚加成反应Polyaddition Reaction聚碳酸酯Polycarbonate, PC聚酯Polyester, PET聚醚Polyether聚合物Polymer聚合物合金Polymer Alloy高分子化学Polymer Chemistry聚苯乙烯Polystyrene, PS聚乙烯Polythene PE聚氨酯Polyurethane聚氯乙烯Polyvinyl Chloride, PVC 几率效应Probable Effect辐射引发Radiation Initiation自由基聚合Radical Polymerization 无规聚合物Random Copolymer无规预聚物Random Prepolymer竞聚率Reactivity Ratios重复单元Repeating Unit缓聚作用Retardation缓聚剂Retarder可逆加成-断裂转移自由基聚合Reversible Addition Fragmentation Transfer, RAFT开环聚合Ring-Opening Polymerization 橡胶Rubber半梯形聚合物Semiladder Polymer侧链Side Chain侧基Side Group固相缩聚Solid Phase Polycondensation 溶液缩聚Solution Polycondensation溶液聚合Solution Polymerization星形聚合物Star-shaped Polymer逐步聚合Step Polymerization立体异构Stereo isomerism有规立构聚合物Stereo-regular Polymer有规立构聚合Stereo-regular Polymerization 定向聚合Stereo-specific Polymerization位阻效应Steric Effect结构单元Structural Unit结构材料Structural Material结构预聚物Structural Prepolymer丁苯橡胶Styrene-Butadiene Rubbers,SBR悬浮聚合Suspension Polymerization增溶胶束Swollen Micelle间规立构Syndiotactic间规度Syndiotacticity间规聚苯乙烯Syndiotactic Polystyrene合成高分子Synthetic Polymer遥爪聚合物T elechelic Polymer涤纶Terylene or Poly(ethylene terephthalate), PET 热引发Thermal Initiation热塑性聚合物Thermoplastic Polymer热固性聚合物Thermosetting Polymer体型缩聚Three Dimensional PolycondensationZigler-Natta引发剂Zigler-Natta -Initiator。
1 A new model of metal plasticity and fracture with pressure and lode dependence(MIT)

A new model of metal plasticity and fracturewith pressure and Lode dependenceYuanli Bai *,Tomasz WierzbickiImpact and Crashworthiness Lab,Massachusetts Institute of Technology,77Massachusetts Avenue,Room 5-218,Cambridge,MA 02139,USAReceived 28June 2007;received in final revised form 28August 2007Available online 14September 2007AbstractClassical metal plasticity theory assumes that the hydrostatic pressure has no or negligible effect on the material strain hardening,and that the flow stress is independent of the third deviatoric stress invariant (or Lode angle parameter).However,recent experiments on metals have shown that both the pressure effect and the effect of the third deviatoric stress invariant should be included in the con-stitutive description of the material.A general form of asymmetric metal plasticity,considering both the pressure sensitivity and the Lode dependence,is postulated.The calibration method for the new metal plasticity is discussed.Experimental results on aluminum 2024-T351are shown to validate the new material model.From the similarity between yielding surface and fracture locus,a new 3D asymmetric fracture locus,in the space of equivalent fracture strain,stress triaxiality and the Lode angle parameter,is postulated.Two methods of calibration of the fracture locus are discussed.One is based on classical round specimens and flat specimens in uniaxial tests,and the other one uses the newly designed but-terfly specimen under biaxial testing.Test results of Bao (2003)[Bao,Y.,2003.Prediction of ductile crack formation in uncracked bodies.PhD Thesis,Massachusetts Institute of Technology]on alumi-num 2024-T351,and test data points of A710steel from butterfly specimens under biaxial testing validated the postulated asymmetric 3D fracture locus.Ó2007Elsevier Ltd.All rights reserved.Keywords:Pressure effect;Lode dependence;Yield surface;Fracture locus;Calibration method0749-6419/$-see front matter Ó2007Elsevier Ltd.All rights reserved.doi:10.1016/j.ijplas.2007.09.004*Corresponding author.E-mail address:byl@ (Y.Bai).Available online at International Journal of Plasticity 24(2008)1071–10961072Y.Bai,T.Wierzbicki/International Journal of Plasticity24(2008)1071–10961.IntroductionThe classical J2theory of metal plasticity assumes that the effect of hydrostatic pressure r m on plasticflow is negligible,and further assumes that theflow stress is independent of the third stress invariant of the stress deviator.For application to ductile fracture,these assumptions must be carefully examined.Ductile fracture is a local phenomenon and the state of stress and strain in potential fracture locations must be determined with great accuracy.Fracture initiation is often preceded by large plastic deformation and there are considerable stress and strain gradients around the point of fracture.In these situations, the infinitesimal J2theory of plasticity is not accurate enough,and more refined plasticity models has to be introduced.The soil and rock mechanics community has long recognized a need for incorporating the hydrostatic and deviatoric(Lode angle parameter)stress invariants into a constitutive descriptions(see for example Bardet,1990;Menetrey and Willam,1995).More recently Bigoni and Piccolroza(2003)proposed a general failure surface for geomaterials in the space of principal stresses that reduces in limiting cases to the Tresca hexagon or the von Mises circle.The Sandia GeoModel(Fossum and Brannon,2006)is also formulated in the space of three invariants.The developments in geomaterials was proceeding over the decades independently of metal plasticity with the latter lagging behind the former.Based on an extensive experimental study,Richmond and Spitzig(Richmond and Spit-zig,1980;Spitzig and Richmond,1984)werefirst to demonstrate the effect of hydrostatic pressure on yielding of aluminum alloys.This conclusion has recently been confirmed by Wilson(2002),who studied notched2024-T351aluminum bars in tension.The concept of a shrinking yield surface with hydrostatic pressure was put forward independently by Gur-son(1975)and later by Needleman and Tvergaard(1984)in their studies of ductile frac-ture by the nucleation,growth and coalescence of voids.The common shortcoming of these various theories of porous plasticity has been an ill-defined calibration procedure.In general,the hydrostatic pressure is controlling the size of the yield surface while the Lode angle parameter is responsible for its shape.The determination of an adequate shape of the yield surface has become an issue in the sheet metal forming industry.It was found a long time ago that the von Mises plane stress ellipse does not lead to a correct prediction of the necking instability.There are an abundance of various modifications and generaliza-tions of the plane stress yield curve to bring the prediction closer to reality(Karafillis and Boyce,1993;Barlat et al.,1991,1997,2005;Vegter and van den Boogaard,2006).How-ever,most of the above theories included the effect of an in-plane anisotropy and the con-nection between the shape of yield condition and the Lode angle parameter has only been noticed recently.In particular,the effect of the Lode angle parameter(or the third devia-toric stress invariant)on plastic yielding has been studied by Cazacu and Barlat(2004), Cazacu et al.(2006),Racherla and Bassani(2007).Their models incorporate the difference in strength under compression and tension.They showed that the forming limit diagram of sheets was sensitive in that difference.However,their models did not have enoughflexibil-ity to predict plane strain yielding.Such a generalization is proposed in the present paper.The paper is divided into two parts.Thefirst part is concerned with a development of a more general plasticity model.Results of21tests on three groups of specimens are ana-lyzed through afinite element simulation.It is shown that the parameters of the J2plas-ticity model with power hardening rule,determined from one test,can not predict correctly the load–displacement responses in the all remaining tests.A new plasticitymodel with correction for the hydrostatic pressure and the Lode parameter brings a per-fect correlation with test results.Percentage-wise,the correction for the hydrostatic pres-sure is small.Physically,it could be attributed to the effect of hydrostatic pressure on metal crystal dislocations.The magnitudes of correction due to the deviatoric state parameter (Lode angle parameter)is large and in some cases reaches20%.The effect of hydrostatic pressure is consistent with earlierfinding of other authors(Richmond and Spitzig,1980; Spitzig and Richmond,1984;Wilson,2002).The new yield surface is asymmetric in the space of principal stresses losing three planes of symmetry as compared to the Tresca yield condition with six plane of symmetry,so it is asymmetric in any of the Tresca’s six sym-metry planes.The second part of the paper deals with the determination of the3D locus of the frac-ture initiation points.The locus is determined experimentally from two types of test pro-cedures.One is based on classical round specimens orflat specimens in uniaxial tests,and the other one uses a series of tests on a double curvature butterfly specimen subjected to bi-axial loading under different combination of tension/shear and compression/shear.The test points are thenfitted to a smooth surface which describes the dependence of the equiv-alent strain to fracture on the average stress triaxiality and the normalized third invariant of the deviatoric stress tensor(or Lode angle parameter).Again,it is shown that the best fit of test data is provided by a surface which is asymmetric with respect to the Lode angle parameter.2.Characterization of the stress stateThe extended metal plasticity model and the3D fracture locus will be formulated in terms of three invariants of the stress tensor[r],defined respectively byp¼Àr m¼À13trð½r Þ¼À13ðr1þr2þr3Þ;ð1Þq¼ r¼ffiffiffiffiffiffiffiffiffiffiffiffiffiffiffiffiffiffi32½S :½Sr¼ffiffiffiffiffiffiffiffiffiffiffiffiffiffiffiffiffiffiffiffiffiffiffiffiffiffiffiffiffiffiffiffiffiffiffiffiffiffiffiffiffiffiffiffiffiffiffiffiffiffiffiffiffiffiffiffiffiffiffiffiffiffiffiffiffiffiffiffiffiffiffiffiffiffiffiffiffiffiffi12½ðr1Àr2Þ2þðr2Àr3Þ2þðr3Àr1Þ2r;ð2Þr¼92½S Á½S :½S1=3¼272detð½S Þ!1=3¼272ðr1Àr mÞðr2Àr mÞðr3Àr mÞ!1=3;ð3Þwhere[S]is the deviatoric stress tensor,½S ¼½r þp½I ;ð4Þ[I]is the identity tensor and r1,r2and r3denote principal stresses.Note that the param-eter p is positive in compression,but r m is positive in tension.It is convenient to work with the dimensionless hydrostatic pressure g,defined byg¼Àpq¼r mr:ð5ÞThe parameter g,often referred to as the triaxiality parameter,has been extensively used in the literature on ductile fracture(McClintock,1968;Rice and Tracey,1969;Hancock and Mackenzie,1976;Mackenzie et al.,1977;Johnson and Cook,1985;Bao,2003).The sec-ond important parameter is the Lode angle h.The geometrical represent of Lode angle is Y.Bai,T.Wierzbicki/International Journal of Plasticity24(2008)1071–10961073shown in Fig.1.The Lode angle h is related to the normalized third deviatoric stress invariant n (see Malvern,1969;Xu and Liu,1995)throughn ¼r q3¼cos ð3h Þ:ð6ÞSince the range of the Lode angle is 06h 6p /3,the range of n is À16n 61.Further-more,the Lode angle h can be normalized byh ¼1À6h p ¼1À2parccos n :ð7ÞThe range of h is À16 h 61.The parameter h will be called the Lode angle parameter hereinafter.Now,all stress directions (or called loading conditions)can be characterized by the above defined set of parameters ðg ; h Þ.Various stress states encountered in ‘‘classi-cal ”specimens used for plasticity and fracture testing can be uniquely characterized by the above defined set of parameters ðg ; h Þ,as shown in Table 1.The analytical expressions for the stress triaxiality g ,the parameter h in terms of measurable quantities are all listed in Table 1.Special attention is given to the plane stress state.It was shown by Wierzbicki and Xue (2005)that the condition r 3=0uniquely relates the parameters g and n (or h )through n ¼cos p 2ð1À h Þh i ¼À272g g 2À13:ð8ÞA plot of Eq.(8)is shown in Fig.2.Points corresponding to 10types of ‘‘classical ”spec-imens and tests are marked by circles.The function h (Eq.(8))has three roots correspond-ing to pure shear (g =0, h ¼0)and the transverse plastic plane strain (g ¼Æ1ffiffi3p , h ¼0).3.A new form of metal plasticityThe plasticity theory to be developed in this section is valid under several assumptions.Firstly,the homogeneity and material isotropy are assumed.Secondly,the material is taken to be elastic–plastic with isotropic hardening.The extension to the combined isotro-pic/kinematic hardening could be made following the procedures suggested by Khan (for example Khan and Jackson,1999).Finally,the plastic incompressibility is assumed.1074Y.Bai,T.Wierzbicki /International Journal of Plasticity 24(2008)1071–10963.1.Effect of hydrostatic pressure on yieldThe concept of pressure dependent yield condition goes back to Coulomb–Mohr,and Drucker and Prager (1952).This concept,originally developed for soil and granular mate-rials,was shown more recently in a number of publications (Richmond and Spitzig,1980;Brownrigg et al.,1983;Spitzig and Richmond,1984;Bru ¨nig,1999)to be applicable to metal plasticity.According to the above theories,the initial and current yield function is taken in this paper to be a linear function of the normalized pressure,g ,r yld ¼ r ð e p ÞÀa I 1¼ r ð e p Þ1À3ar m p !¼ r ð e p Þð1À3ag Þ;ð9ÞTable 1Ten types of classical specimens for plasticity and fracture calibrationNo.Specimen type Analytical expressions for stress triaxiality g a The Lode angle parameter h 1Smooth round bars,tension 1312Notched round bars,tension (Bridgman,1952)13þffiffiffi2p ln 1þa 2R ÀÁ13Plastic plane strain,tension ffiffi3p 304Flat grooved plates,tension (Bai et al.,2006b )ffiffi3p 31þ2ln 1þt 4R ÀÁÂÃ05Torsion or shear 006Cylinders,compression À13À17Equi-biaxial plane stress tension 23À18Equi-biaxial plane stress compression À2319Plastic plane strain,compression Àffiffi3p 3010Notched round bars,compression À13þffiffiffi2p ln 1þa 2R ÀÁÂÃÀ1a In the expressions of g ,R is the radius of a notch or a groove,a is the radius of a round bar at the notch,t is the thickness of a flat grooved plate at the groove.Y.Bai,T.Wierzbicki /International Journal of Plasticity 24(2008)1071–109610751076Y.Bai,T.Wierzbicki/International Journal of Plasticity24(2008)1071–1096where I1=3r m and e p is the equivalent plastic strain.The proportionality parameter a should be calibrated from tests.In Eq.(9),thefirst strain hardening term, rð e pÞ,represents the stress–strain curve in zero hydrostatic pressure,for example in the torsion test.In prac-tice,tensile tests of a smooth round bar or a dog-bone specimen are very commonly used to calibrate the stress–strain curve.Therefore,more generally,Eq.(9)can be rewritten asr yld¼ rð e p;g Þ½1À3aðgÀg Þ ¼ rð e pÞ½1Àc gðgÀg Þ ;ð10Þwhere rð e pÞis material strain hardening function from the reference test,and g is the ref-erence value of stress triaxiality from the reference test,for example,g =1/3for smooth round bar tensile test,g =À1/3for cylindrical specimen compressive test,g =0for tor-sion test and so on.Here,c g is a material constant need to be calibrated,which represents the hydrostatic pressure effect on material plasticity.It should also be noted that the effect of pressure on plasticity does not have to be linear.Experimental data for ices showed a non-linear pressure effect(Karr et al.,1989).A linear function is proposed in this paper for metals.The present concept of pressure dependent yield function for metals should not be con-fused with Gurson type of softening(Gurson,1975;Needleman and Tvergaard,1984)due to the nucleation,growth and linkage of voids.In a simple tensile test,it is difficult to tell what physical mechanism is responsible for the observed softening-the suppression of dis-location motion or the growth of material porosity with deformation.The physically based Eq.(10)explains a relative difference in the stress–strain curves obtained in tests with various stress triaxialities,which can be measured experimentally by a few simple tests.At the same time,the material softening in the GTN model(Needleman and Tverg-aard,1984)is an illusive concept because the reference state is the undamaged stress–strain curve for the matrix material,which can not be found from any simple tests.The revers-ibility of the softening is another issue even though(Spitzig and Richmond,1984)did not address it directly.It is implicitly assumed that the dislocation suppression is reversible. This means that when in the loading process the hydrostatic pressure is brought back to the original value,the material will regain its initial strength.It is useful to design a test program involving a controlled change of the triaxiality to distinguish the two types of softening as discussed above.One such test could,for example,would involve two-stage tensile tests on notched round bars.After some plastic deformation the test is stopped and the gauge section of a specimen is re-machined to a larger radius.Such tests are being planned by the present authors.3.2.Lode dependenceThe dependence of the yield condition on the Lode angle parameter can best be explained by comparing the von Mises and Tresca yield condition.In the polar coordinate system,the equivalent stress becomes the radial coordinate while the circumferential coor-dinate is the Lode angle h.In the deviatoric stress plane,the von Mises yielding condition is represented by a circle.The Tresca yielding is a hexagon inscribed on the von Mises cir-cle,as shown in Fig.3.To take the third deviatoric stress invariant into account,a new term considering the effect of the Lode angle is introduced into Eq.(10).The following yield criterion is proposed:r yld ¼ r ð e p Þ½1Àc g ðg Àg 0Þ c s h þðc ax h Àc s h Þc Àc m þ1m þ1 !;ð11Þwhere c and c ax h are two parameters defined by c ¼cos ðp =6Þ1Àcos ðp =6Þ1cos ðh Àp =6ÞÀ1 !¼6:4641½sec ðh Àp =6ÞÀ1 ;ð12Þc ax h ¼c t h for h P 0;c c h for h <0:(ð13ÞEq.(11)defines a class of function that define the shape of the yield surface.The term cos(h Àp /6)in the definition of the parameter c represents the difference between von Mises and Tresca in the deviatoric stress plane,which is obtained from the geometrical construction by comparing the von Mises circle and the Tresca hexagon.After modifica-tion and normalization,the range of c is 06c 61,in which c =0is corresponding to plane strain or shear condition,and c =1is corresponding to axial symmetry.In Eq.(11),the leading term is linear with respect to the parameter c ,the higher order power term c m þ1m þ1 is introduced to make the yield surface smooth and differentiable with respect to Lode angle h around c =1.The parameter m is a non-negative integer.There are fourmaterial constants,c t h ,c s h ,c c h and m ,need to be calibrated.The values of c t h ,c s h ,and c c h are relative,and at least one of them is equal to unity.This depends on which type of ref-erence test is used to calibrate the strain hardening function rð p Þ.For example,if a smooth round bar tensile test is used,then c t h ¼1;if a torsion test is used,then c s h ¼1;if a cylinder specimen compressive test is used,then c c h ¼1.It can be proved that the postulated yield function (Eq.(11))is smooth and differentia-ble.The convexity of yield surface is controlled by the ratios of three parameters c t h ,c c h andc s h .Compared with von Mises and Tresca yield conditions,this yield surface can be plotted in the deviatoric stress plane,as shown in Fig.3.Examples of yield loci are shown in Fig.4for the case of plane stress.By suitably choosing model parameters,some limiting cases are obtained.For example,assuming either c g =0and c t h ¼c s h ¼c c h ¼1or m =0gives the von Mises yield condition,Y.Bai,T.Wierzbicki /International Journal of Plasticity 24(2008)1071–10961077while taking c g =0and c s h ¼ffiffiffi3p =2;c t h ¼c c h ¼1;m ¼þ1gives the Tresca yield criterion.If the parameter c g ¼0in above two cases,then one will get Drucker and Prager yield function (1952)and pressure-modified Tresca yield function.Most of metal plasticity the-ories assume that,in the deviatoric stress plane,the yield function is symmetric for uniaxial tension and uniaxial compression,which are corresponding to h ¼1and h ¼À1respec-tively (for example,Tresca,1864;Mises,1913;Barlat et al.,1991;Karafillis and Boyce,1993).This restriction can be removed in the present model by setting c t h ¼c c h .It shouldbe noted that the flattening property of the von Mises ellipse,which is needed for right prediction of the Forming Limit Curves,was introduced by many authors in the space of principal stresses using non-quadratic yield function (Hosford,1972;Karafillis and Boyce,1993;Barlat et al.,1991,1997),often under plane stress assumption.Vegter and van den Boogaard (2006)modeled the plane stress yield locus by fitting Brezier curves into four test points.Working on the deviatoric plane with the Lode angle dependence is another way of controlling the shape of the yield surface in a simple and elegant way.3.3.The deviatoric associated flow ruleThe yield surface,given by Eq.(11),can be visualized in the space of three principle stresses (r 1,r 2,r 3),see Fig.5.According to the conventional associated flow rule,d e plij ¼d k o f o r ij ;ð14Þwhere the plastic potential is defined byf ¼q À r ð e p Þ½1Àcg ðg Àg 0Þ c sh þðc ax h Àc s h Þc Àc m þ1m þ1 !;ð15ÞFig.4.Examples of yield locus for plane stress condition.(Here,c t h ¼1,and the effect of hydrostatic pressure is dis-activated,c g =0).1078Y.Bai,T.Wierzbicki /International Journal of Plasticity 24(2008)1071–1096d k is the equivalent plastic strain increment,and q is defined by Eq.(2).To implement a yield function intofinite element codes,a necessary step is to derive the expression for thenormal direction(orflow direction)with respect to yield locus,o fij .From the yield func-tion,Eq.(15),one can geto f o r ij ¼o qo r ijþ rð e pÞc g c shþðc axhÀc shÞcÀc mþ1mþ1!o go r ijÀ rð e pÞ½1Àc gðgÀg ÞÂðc axhÀc shÞð1Àc mÞo co r ij;ð16Þwhere o qo r ij ,o go r ijand o co r ijcan be expressed by the following equations.o q o r ij ¼32qs ij;ð17Þo g o r ij ¼13qd ijÀ3g2q2s ij;ð18Þo c o r ij ¼3ffiffiffi3p2Àffiffiffi3p!tanðhÀp=6ÞcosðhÀp=6Þ1q sin3hd ij3þcos3h2qs ijÀ32q2s ik s kj:ð19ÞAlthough the plasticity is pressure sensitive,experiments show that the plastic dilatancy of metals is negligible(Spitzig and Richmond,1984).To satisfy the assumption of plasticincompressibility,thefirst term13q d ij in Eq.(18)should be removed.Therefore,what isused in the present paper is aflow rule with deviatoric associativity,see Eq.(20),rather than the conventional associatedflow rule,Eq.(16).d e pl ij¼d k32qs ijÀ rð e pÞc g c shþðc axhÀc shÞcÀc mþ1mþ1!3g2q2s ij &À rð e pÞ½1Àc gðgÀg Þ ðc axh Àc shÞð1Àc mÞÂ3ffiffiffi3p2Àffiffiffi3p!tanðhÀp=6ÞcosðhÀp=6Þ1q sin3hd ij3þcos3h2qs ijÀ32q2s ik s kj):ð20ÞFig.5.A postulated3D yield surface.Y.Bai,T.Wierzbicki/International Journal of Plasticity24(2008)1071–10961079Clearly,the presence of additional terms in Eq.(20)indicate that the direction of plastic flow is normal to the yield surface in the deviatoric plane but not to von Mises yield sur-face.In this issue,the present formulation can be classified as an intermediateflow rule between the non-associatedflow rule and the conventional‘‘fully”associated one.(It should be noted that if this model is used to model other non-metallic porous materials, which are plastic compressible,then a fully associatedflow rule as previous stated is nec-essary).This new form of metal plasticity has been implemented into Abaqus user material subroutine VUMAT using the classical mapping return algorithm.The following numer-ical simulations are run with the material subroutine.4.Experimental calibration of2024-T351aluminumA power function is introduced to describe the isotropic stain hardening for metal plasticity.rð e pÞ¼Aðe þ e pÞn for r P r y;ð21Þwhere is thefirst yield strain.There are nine parameters,A, ,n,c g,g ,c th ,c sh,c ch,and m,in the present plasticity model.To validate and calibrate the model,four types of tests are required.Thefirst test is the smooth round bar tensile test,from which the baseline stress–strain curve(parameters:A, and n)can be determined.Also,the reference parametersare uniquely defined:g =1/3and c th ¼1.The second test is the notched round bars tensiletest.Introducing a geometric notch to a smooth round bar increases the hydrostatic pres-sure in the materials inside the neck.Wilson(2002)conducted this type of test on alumi-num2024-T351,and calibrated the pressure effect on metal plasticity,but the effect of Lode angle was not taken into account.The third test is the tensile test offlat groovedplates,which can be used to calibrate the parameter c sh .The fourth test is the cylindricalspecimens’upsetting test,which can be used to calibrate the last two parameters c ch andm.The corresponding stress states of these four types of tests are also respectively marked as A,B,C and D in Fig.3.The analysis and a description of experimental study of thefirst three types of tests were described in the earlier report(Bai et al.,2006b)in detail.In this paper,emphasis is put on the numerical simulation study and plastic model calibration.4.1.Smooth and notched round bars tensile testsSmooth round bar tensile tests were used to calibrate the stress–strain curve.There are three steps involved.Firstly,the engineering stress–strain curve r E(e E)is obtained from the force–displacement curve P(u)recorded during the test.Secondly,the true stress–strain curve rð eÞis calculated from the engineering stress–strain curve using the classical trans-formation law, r¼r Eð1þe EÞand e¼lnð1þe EÞ.In this step,only the data point before specimen necking can be used because the transformation equations are valid only up to necking initiation.Approximately,one can take the peak point of the force–displacement curve as the necking initiation point.Thirdly,a power function(Eq.(21))is used tofit the true stress–strain curve obtained from the test.This curve is then extrapolated to get the approximate true stress–strain curve after necking.Two methods are available to determine more precisely the true stress–strain curve after necking.If the continuous measurements of the neck geometry are available,then the Bridgman correction is applicable.Alternatively,the inverse method could be used in 1080Y.Bai,T.Wierzbicki/International Journal of Plasticity24(2008)1071–1096which the stress–strain curve after necking is adjusted to get good agreement of the mea-sured force–displacement curve.For the present material,the neck was not deep and it was not necessary to adjust the stress–strain curve after necking.A group of tensile tests of smooth and notched round bars were conducted,refer to Fig.6.The diameter of smooth round bar specimens is9mm.For notched round bar spec-imens,the diameter of the minimal cross-section is8mm,and two radii of notches are assigned,equal to12mm and4mm,respectively.The diameters in specimens shoulder are all equal to15mm,and the length of gauge section for this group of specimens is 25.5mm.The selected material is aluminum2024-T351.To diminish a possible effect of material anisotropic,all the specimens discussed in this paper are machined from the roll-ing direction(or90°direction)of a same material block.Furthermore,Bao(2003)has shown that the effect of anisotropy of2024-T351aluminum alloy is negligible.Two to four tests are conducted for each case.The stress–strain curve was calibrated from a smooth round bar tensile test,so g =1/3. To calibrate the pressure dependence coefficient c g,thefinite element models of the spec-imens were constructed with the Lode angle terms switched off.Round bar specimens are discretized by4-node axisymmetric elements.Finite element models of Abaqus/explicit are built,in which the user material subroutine VUMAT is used.The measured force–dis-placement curves are shown in Figs.7and8.In order to study possible mesh dependency, calculations were run usingfine(0.1mm)and coarse(0.2mm)meshes.The maximal dif-ference of the force–displacement response was less than0.5%,meaning that thefinite ele-ment solution was convergent.All the results presented in this paper use thefine mesh size. From Figs.7and8,one can see that without any corrections,the force–displacement response of the smooth round bar simulation agrees with the experimental results very well,but the notched round bar simulations over-predict the force–displacement curves about3–6%.Similar reduction in the material strength were also reported by Bru¨nig (1999)and Kuroda(2004).The reason is that the material points inside a notched round bar are subjected to higher pressure than the smooth round bar.This phenomenon shows the effect of hydrostatic pressure on stress–strain curve.By changing the coefficient c g iteratively,a value of c g=0.09is obtained,which makes the force–displacement curvesFig.6.A group of smooth and notched round bar specimens.。
Review on
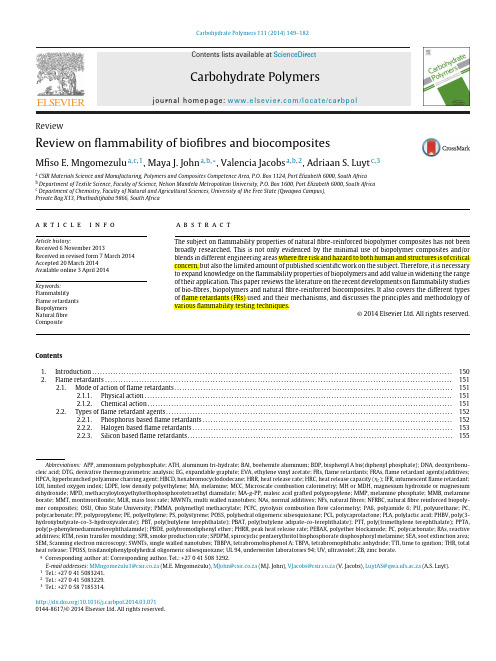
Carbohydrate Polymers 111(2014)149–182Contents lists available at ScienceDirectCarbohydratePolymersj o u r n a l h o m e p a g e :w w w.e l s e v i e r.c o m /l o c a t e /c a r b p olReviewReview on flammability of biofibres and biocompositesMfiso E.Mngomezulu a ,c ,1,Maya J.John a ,b ,∗,Valencia Jacobs a ,b ,2,Adriaan S.Luyt c ,3aCSIR Materials Science and Manufacturing,Polymers and Composites Competence Area,P.O.Box 1124,Port Elizabeth 6000,South Africa bDepartment of Textile Science,Faculty of Science,Nelson Mandela Metropolitan University,P.O.Box 1600,Port Elizabeth 6000,South Africa cDepartment of Chemistry,Faculty of Natural and Agricultural Sciences,University of the Free State (Qwaqwa Campus),Private Bag X13,Phuthaditjhaba 9866,South Africaa r t i c l ei n f oArticle history:Received 6November 2013Received in revised form 7March 2014Accepted 20March 2014Available online 3April 2014Keywords:Flammability Flame retardants Biopolymers Natural fibre Compositea b s t r a c tThe subject on flammability properties of natural fibre-reinforced biopolymer composites has not been broadly researched.This is not only evidenced by the minimal use of biopolymer composites and/or blends in different engineering areas where fire risk and hazard to both human and structures is of critical concern,but also the limited amount of published scientific work on the subject.Therefore,it is necessary to expand knowledge on the flammability properties of biopolymers and add value in widening the range of their application.This paper reviews the literature on the recent developments on flammability studies of bio-fibres,biopolymers and natural fibre-reinforced biocomposites.It also covers the different types of flame retardants (FRs)used and their mechanisms,and discusses the principles and methodology of various flammability testing ©2014Elsevier Ltd.All rights reserved.Contents 1.Introduction (150)2.Flame retardants ....................................................................................................................................1512.1.Mode of action of flame retardants..........................................................................................................1512.1.1.Physical action .....................................................................................................................1512.1.2.Chemical action ....................................................................................................................1512.2.Types of flame retardant agents .............................................................................................................1522.2.1.Phosphorus based flame retardants ...............................................................................................1522.2.2.Halogen based flame retardants ...................................................................................................1532.2.3.Silicon based flame retardants.....................................................................................................155Abbreviations:APP,ammonium polyphosphate;ATH,aluminum tri-hydrate;BAl,boehemite aluminum;BDP,bisphenyl A bis(diphenyl phosphate);DNA,deoxyribonu-cleic acid;DTG,derivative thermogravimetric analysis;EG,expandable graphite;EVA,ethylene vinyl acetate;FRs,flame retardants;FRAs,flame retardant agents/additives;HPCA,hyperbranched polyamine charring agent;HBCD,hexabromocyclododecane;HRR,heat release rate;HRC,heat release capacity (Ác );IFR,intumescent flame retardant;LOI,limited oxygen index;LDPE,low density polyethylene;MA,melamine;MCC,Microscale combustion calorimetry;MH or MDH,magnesium hydroxide or magnesium dihydroxide;MPD,methacryloyloxyethylorthophosphorotetraethyl diamidate;MA-g -PP,maleic acid grafted polypropylene;MMP,melamine phosphate;MMB,melamine borate;MMT,montmorillonite;MLR,mass loss rate;MWNTs,multi walled nanotubes;NAs,normal additives;NFs,natural fibres;NFRBC,natural fibre reinforced biopoly-mer composites;OSU,Ohio State University;PMMA,polymethyl methacrylate;PCFC,pyrolysis combustion flow calorimetry;PA6,polyamide 6;PU,polyurethane;PC,polycarbonate;PP,polypropylene;PE,polyethylene;PS,polystyrene;POSS,polyhedral oligomeric silsesquioxane;PCL,polycaprolactone;PLA,polylactic acid;PHBV,poly(3-hydroxybutyrate-co-3-hydroxyvalerate);PBT,poly(butylene terephthalate);PBAT,poly(butylene adipate-co-terephthalate);PTT,poly(trimethylene terephthalate);PPTA,poly(p-phenylenediamineterephthalamide);PBDE,polybromodiphenyl ether;PHRR,peak heat release rate;PEBAX,polyether blockamide;PC,polycarbonate;RAs,reactive additives;RTM,resin transfer moulding;SPR,smoke production rate;SPDPM,spirocyclic pentaerythritol bisphosphorate disphosphoryl melamine;SEA,soot extinction area;SEM,Scanning electron microscopy;SWNTs,single walled nanotubes;TBBPA,tetrabromobisphenol A;TBPA,tetrabromophthalic anhydride;TTI,time to ignition;THR,total heat release;TPOSS,trisilanolphenylpolyhedral oligomeric silsesquioxane;UL 94,underwriter laboratories 94;UV,ultraviolet;ZB,zinc borate.∗Corresponding author at:Corresponding author.Tel.:+270415083292.E-mail addresses:MMngomezulu1@csir.co.za (M.E.Mngomezulu),MJohn@csir.co.za (M.J.John),VJacobs@csir.co.za (V.Jacobs),LuytAS@qwa.ufs.ac.za (A.S.Luyt).1Tel.:+270415083241.2Tel.:+270415083229.3Tel.:+270587185314./10.1016/j.carbpol.2014.03.0710144-8617/©2014Elsevier Ltd.All rights reserved.150M.E.Mngomezulu et al./Carbohydrate Polymers111(2014)149–1822.2.4.Nano metric particles (155)2.2.5.Mineralflame retardant (159)3.Flammability testing techniques (161)3.1.Cone calorimetry (161)3.2.Pyrolysis combustionflow calorimetry(PCFC) (162)3.3.Limiting oxygen index(LOI) (163)3.4.UnderwritersLaboratories94(UL94) (164)3.5.Ohio State University heat release apparatus(OSU) (164)4.Flammability of biofibres and biocomposites (166)4.1.Biofibres(naturalfibres) (166)4.2.Biopolymers (171)4.3.Biofibre reinforced biopolymer composites (175)5.Summary (178)Acknowledgements (179)References (179)1.IntroductionIn recent years,the research on biofibre reinforced biopolymer composites has advanced.This development is motivated by factors such as shortage of and high fossil energy cost,and the current shift towards environmentally tolerant or“green”composite materials. The shift towards environmentally friendly biocomposite materi-als is due to environmental legislation,the REACH Act(Registration, Evaluation,Authorization and Restriction of Chemical substances), comparable properties to syntheticfibre counterparts,green attri-bution and low cost.Most of the components in biocomposites are based on agricultural products as a source of raw materials.Thus, their use provides solution for waste disposal,reduction in agricul-tural residues and hence environmental pollution resulting from the burning of these.Additionally,it offers an economical solution for farming and rural areas in developing countries(Anandjiwala et al.,2013;Chapple&Anandjiwala,2010;Faruk,Bledzki,Fink,& Sain,2012;Horrocks,2011;Jang,Jeong,Oh,Youn,&Song,2012; John&Thomas,2008;Kandola,2012;Sahari&Sapuan,2011; Satyanarayana,Arizaga,&Wypych,2009).Biofibre reinforced biopolymer composite materials largely have appealing properties.They are renewable,recyclable(par-tially or completely),relatively cheap,biodegradable and thus environmentally friendly.However,there are some inherent dis-advantages such as their hydrophilic nature and poorflammability properties(i.e.poorfire resistance).The attractive properties clearly outweigh the undesirable ones and the latter have remedial measures.For example,remedies may be chemical and/or phys-ical modifications such as the incorporation offlame retardant additives(FRAs)to improveflammability of biocomposites(John &Thomas,2008).Previous research observed limitations in the use of biofibre reinforced biopolymer composites,especially in areas that posefire hazard and risk.This is because naturalfibre reinforced biopoly-mer composites are largely used in the packaging and automotive industries wherefire safety regulatory requirements are not as stringent as those in the aerospace industry.Therefore,to broaden the range of applications of these biocomposites into other sec-tors of advanced engineering(i.e.aerospace,marine,electronics equipment and construction),both theirflammability characteris-tics andfire retardance strategies need more research(Bourbigot& Fontaine,2010;Chapple&Anandjiwala,2010;Kandola,2012).There are different strategies that can be demonstrated forfire retardancy of biocomposites.Fire retardancy is the phenomenon in which materials such as plastics and/or textiles are rendered less likely ignitable or,if they are ignitable,should burn with less efficiency(Price,Anthony,&Carty,2001).It may be achieved by use of several approaches.These may be chemical modification of existing polymers,addition of surface treatment to the polymers,use of inherentlyfire resistant polymers or high performance poly-mers,and direct incorporation offlame retardants(FRs)and/or micro or nanoparticles in materials.The direct incorporation of flame retardants is achieved through use of various additives.These flame retardance strategies may range from the use of phospho-rus additives(e.g.intumescent systems),halogen additives(e.g. organobromine),silicon additives(e.g.silica),nanometric particles (e.g.nanoclays)and minerals based additives(e.g.metal hydrox-ide).The broader information onflame retardant additives(FRAs) in natural polymers,wood and lignocellulosic materials has been reviewed by Kozlowski and Wladyka-Przybylak(2001).Thus,the primary duty offlame retardant systems is to prevent,minimize, suppress or stop the combustion of a material(Laoutid,Bonnaud, Alexandre,Lopez-Cuesta,&Dubois,2009;Morgan&Gilman,2013; Price et al.,2001;Wichman,2003).Flame retardant systems can either act chemically or physically in the solid,liquid or gas phase.These mechanisms are dependent on the nature of theflame retardant system.The chemical mode of action may be manifested by reaction in the gaseous and condensed phases,whereas the physical mode occurs by a cooling effect,for-mation of a protective layer or by fuel dilution.FRs may be classified into three classes.They are normal additives(NAs),reactive addi-tives(RAs)and a combination of FRs(Laoutid et al.,2009;Price et al.,2001;Wichman,2003).Theflammability offire retarded materials may be tested through differentfire testing techniques.The most widely used laboratoryflammability testing techniques have been reported in literature(Laoutid et al.,2009;Price et al.,2001;Wichman,2003).A number of small,medium and full scaleflammability tests are used in both academic and industrial laboratories.They are employed for either screening the materials during production or testing the manufactured products.These techniques are cone calorime-try,pyrolysis combustionflow calorimetry(PCFC),limiting oxygen index(LOI),and underwriters’laboratories94(UL94)and Ohio State University(OSU)heat release rate tests.These techniques involve the measurement of variousflammability parameters by appropriate tests depending on the targeted application of a poly-meric material.Theflammability of polymers can be characterized by parameters such as ignitability(ignition temperature,delay time,critical heatflux),burning rates(heat release rate,solid degra-dation rate),spread rates(flame,pyrolysis,and smoulder),product distribution(emissions of toxic products)and smoke production (Carvel,Steinhaus,Rein,&Torero,2011;Laoutid et al.,2009;Price et al.,2001).Theflammability properties of naturalfibrereinforced biopoly-mer composites have not been studied extensively.The aim of this paper is to review the current research and developments related toflammability of biofibre reinforced biopolymer composites for the period2000–2013.This review will explore aspects such as theM.E.Mngomezulu et al./Carbohydrate Polymers111(2014)149–182151different types offlame retardants,laboratoryflammability testing techniques and recent studies onflammability of biopolymers and biocomposites.2.Flame retardantsFRs impartflame retardancy character to materials such as coatings,thermoplastics,thermosets,rubbers and textiles.These FRs may prevent,minimize,suppress or stop the combustion pro-cess of materials.They act to break the self sustaining polymer combustion cycle shown in Fig.1,and consequently reduce the burning rate or extinguish theflame in several ways(Guillaume, Marquis,&Saragoza,2012;Grexa&Lübke,2001;Kandola,2001; Kandola&Horrocks,2001;Ke et al.,2010;Kozlowski&Wladyka-Przybylak,2001;Laoutid et al.,2009;Morgan&Gilman,2013;Price et al.,2001;Wichman,2003).The possible ways to reduce the burning rate or extinguish theflames are:(i)the modification of the pyrolysis process in order to lower the quantity of evolvedflammable volatiles,with normally an increase in the formation of char(lessflammable)serv-ing as barrier between the polymer andflame(stage‘a’,Fig.1); (ii)the isolation of theflame from the oxygen/air supply(stage ‘b’);(iii)introduction into the polymer formulations those com-pounds that will release efficientflame inhibitors(e.g.chlorine and bromine)(stage‘c’);and(iv)the lowering of thermal feedback to the polymer to prevent further pyrolysis(stage‘d’)(Price et al., 2001).Toflame retard polymer materials or to protect them fromfire, there are three main approaches to be considered.These are the engineering approach,use of inherently lowflammable polymers and the use offlame retardant additives(FRAs)(Morgan&Gilman, 2013).The engineering approach is cost effective and relatively easy to implement.It requires the use of afire protection shield.However, the method has some limitations such as tearing and/or ripping off(offire proof fabric),loss of adhesion(in metalfire protection), and scratching away and falling off due to impact or ageing(of intumescent paint).Consequently,the underlying material may be left exposed tofire damage.The inherentlyflame retarded polymers can be made in various forms and are easy to implement in different applications.Their use,though,can be limited by high cost and difficulty to recycle(i.e.fibre reinforced polymer composites).As a result,lowflammability polymers are less used except for applications demanding their use (e.g.aerospace and military sectors).The use of FRAs is a well known approach,cost effective and relatively easy to incorporate into polymers.The challenges with this approach,however,include potential for leaching into envi-ronment,difficulty with recycling and a compromise in reaching a balance in properties of a polymer.Regardless of these problems, FRAs are still used.FRs are classified into three categories.They are normal additives(NAs)flame retardants,reactive additives(RAs)flame retardants and combinations of FRs.NAs are incorporated during polymerization or during melt mixing processing and react with the polymer only at higher temperatures at the start of afire.They are commonflame retardant additives and their interaction is physical with the substrate.NAs usually include mineralfillers,hybrids or organic compounds that can include macromolecules.RAs,on the other hand,are usually introduced into polymers during polymer-ization or in a post reaction process.During polymerization,RAs are introduced as monomers or precursor polymers whereas in a post reaction process their introduction is by chemical grafting.These flame retardants chemically bond to the polymer -binations of NAs and RAs can produce an additive(sum),synergistic (higher)or antagonistic(lower)effect.A synergistic effect typically occurs when they are used together with specificflame retardants (Kozlowski&Wladyka-Przybylak,2001;Morgan&Gilman,2013; Price et al.,2001;Troitzsch,1998).2.1.Mode of action offlame retardantsFlame retardant systems can act either chemically or physi-cally in the solid,liquid or gas phase.Such actions do not occur singly but should be considered as complex processes in which var-ious individual stages occur simultaneously,with one dominating. They are dependent on the nature offlame retardant system in place(Bourbigot&Duquesne,2007;Laoutid et al.,2009;Morgan& Gilman,2013;Price et al.,2001;Troitzsch,1998;Wichman,2003). Various modes offlame retardants are discussed in subsequent sections.2.1.1.Physical actionThe physical mode occurs by(i)cooling effect,(ii)fuel dilu-tion or(iii)via formation of a protective layer(coating)(Chapple& Anandjiwala,2010;Jang,Jeong,Oh,Youn,&Song,2012;Kandola, 2012;Laoutid et al.,2009;Price et al.,2001;Troitzsch,1998; Wichman,2003).(i)Cooling effect:Some FRAs(e.g.hydrated trialumina and mag-nesium hydroxide)decompose by an endothermic process and trigger temperature decrease in the system.Cooling of the medium to below the polymer combustion temperatures is effected.Such endothermic reaction is known to act as a heat sink.(ii)Fuel dilution:During decomposition offlame retardants(e.g.aluminum hydroxide),the formation of gases such as H2O,CO2, and NH3lead to dilution of the mixture of combustible gases.Consequently,this limits both the concentration of reagents and the possibility of materials to ignite.(iii)Formation of a protective layer(coating):Some FRAs(e.g.phos-phorus and boron compounds)form a protective solid or gaseous layer between the gaseous and solid combustible phases.This limits the transfer of combustible volatile gases, excludes oxygen necessary for combustion and thus reducing the amount of decomposition gases.2.1.2.Chemical actionThe chemical mode of action may be manifested by reaction in the(i)gaseous and(ii)condensed phase(Chapple&Anandjiwala, 2010;Jang et al.,2012;Kandola,2012;Laoutid et al.,2009;Price et al.,2001;Troitzsch,1998;Wichman,2003).(i)Gaseous phase:By incorporation of FRAs that favour the releaseof specific radicals(e.g.halogenflame retardants,Cl•and Br•) in the gas phase,the free radical mechanism of the combustion process can be stopped.These radicals can react with highly reactive species such as H•and OH•to form less reactive or inert molecules.The exothermic reactions are then stopped;the system cools down and the supply offlammable gases is subsequently reduced.(ii)Condensed phase:Two types of chemical reaction initiated by FRAs are possible:(a)flame retardants can speed up the rupture of polymer chains and the polymer will drip,thus moving away from theflame action zone;(b)FRs can cause the formation ofa carbonized or vitreous layer at the surface of the polymer.This occurs by chemical transformation of degraded polymer chains.The formed char and/or vitreous layer acts as a physical insulating barrier between the gas and condensed phases.152M.E.Mngomezulu et al./Carbohydrate Polymers 111(2014)149–182Fig.1.Demonstration of the self-sustaining polymer combustion cycle;a–d represent potential modes of flame retardants.Adapted from Price et al.(2001).2.2.Types of flame retardant agentsFRAs are based on various chemical compounds.This subsection discusses chemical compounds based on phosphorus,halogen,sil-icon,nanometric particles and mineral additives.The phosphorus based additives include organic phosphorus,inorganic phospho-rus,red phosphorus and intumescent flame retardant systems.The silicon based additives consist of silica and silicones,the nanomet-ric particles based ones may be carbon nanotubes,nanoclays and nanoscale particulate additives,and the minerals based flame retar-dant additives are hydrocarbonates,metal hydroxides and borates.2.2.1.Phosphorus based flame retardantsPhosphorus based FRs include phosphorus into their structure.Their structure can vary from inorganic to organic forms,and with oxidation states of 0,+3,or +5.Phosphorus based FRs consist of phosphates,phosphonates,phosphinates,phosphine oxide and red phosphorus.These FRAs are used as NAs or RAs incorporated into the polymer chain during synthesis.They are effective with oxy-gen or nitrogen containing polymers (cellulose,polyesters,and polyamides).Phosphorated FRs are unique in that they can be con-densed phase or vapour phase FRs depending on their chemical structure and interaction with the polymer under fire conditions (Faruk et al.,2012;Jang et al.,2012;Laoutid et al.,2009).In the condensed phase ,their thermal decomposition leads to the production of phosphoric acid that readily condenses to give phosphorylated structures and gives off water.Released water dilutes the oxidizing gas phase (physical action:fuel dilution).Addi-tionally,phosphoric acid and pyrophosphoric acid can facilitate a dehydration reaction resulting in the formation of carbon to carbon double bonds and charring.This can then lead to the generation of crosslinked or carbonized structures at high temperatures (Faruk et al.,2012;Jang et al.,2012;Laoutid et al.,2009;Morgan &Gilman,2013;Troitzsch,1998).At high temperatures both ortho and pyrophosphoric acid are turned into metaphosphoric acid (OPOOH)and their corresponding polymers (PO 3H)n .Phosphate anions (pyro and polyphosphates)then partake in char formation (with carbonized residue).This car-bonized layer isolates and protects the polymer from the flames,limits the volatilization of fuel,prevents formation of new free radicals,limits the diffusion of oxygen thus reducing combustion,and insulates the polymer underneath from the heat (Faruk et al.,2012;Jang et al.,2012;Laoutid et al.,2009;Morgan &Gilman,2013;Troitzsch,1998).Phosphorus based flame retardants can also volatilize into vapour phase forming active radicals (PO 2•,PO •and HPO •)and act-ing as scavengers of H •and OH •radicals.Volatile phosphorated compounds are among the effective inhibitors of combustion com-pared to bromine and chlorine radicals.Since phosphorus based flame retardants are significantly effective in oxygen and nitrogen containing polymers,it is thus important to have these atoms in the polymer chain.In case the used polymer lacks these atoms in its chain and cannot contribute to charring,a highly charring coadditive {e.g.polyol (pentaerythritol)}has to be introduced in combination with the phosphorated flame retardant.Polymers such as polyamides and polyurethane can also be used as char-ring agents in intumescent flame retardant systems (Faruk et al.,2012;Jang et al.,2012;Laoutid et al.,2009;Morgan &Gilman,2013;Troitzsch,1998).anic phosphorus.Many organic phosphorus derivatives show flame retardancy properties.But,those of commercial impor-tance are limited by the processing temperature and the nature of the polymer to be modifianic phosphorus based FRs can act as NAs or as RAs monomers or co monomers/oligomers.Their main groups are phosphate esters,phosphonates and phosphinates.Due to their high volatility and relatively low fire retardant efficiency,the use of alkyl substituted triaryl phosphate (i.e.triphenyl phos-phate,TPP,cresyl diphenyl phosphate,isopropylphenyl diphenyl phosphate,tertbutylphenyl diphenyl phosphate or tricresyl phos-phate)is limited in plastics engineering.Oligomeric phosphates with lower volatility and higher thermal stability than triaryl phos-phate can be used for plastics engineering.These may be resorcinol bis(diphenyl phosphate)(RDP)and bisphenol A bis(diphenyl phosphate (BDP).The combination of volatile and nonvolatile phosphates can also lead to a synergistic effect.This may be a positive combination of the condensed phase and gas phase of phosphates.The use of reactive phosphorus flame retardants isM.E.Mngomezulu et al./Carbohydrate Polymers111(2014)149–182153also a solution for avoiding volatilization during thermal decompo-sition and migration towards the surface of a polymer.They can be incorporated directly within the polymer chain structure and can be used either as monomers for copolymerization with one or two co-monomers to get phosphorated polymers or as oligomers that react with polymers to form branched or grafted phosphorated polymers(Faruk et al.,2012;Jang et al.,2012;Laoutid et al.,2009; Morgan&Gilman,2013).2.2.1.2.Inorganic phosphorus.A typical example of an inorganic phosphorus salt is a combinationof polyphosphoric acid and ammonia called ammonium polyphosphate(APP).It is either a branched or unbranched polymeric compound with variable chain length(n).For short and linear chain APPs(where n is less than100, crystalline form I),they are more water sensitive and less thermally stable,whereas APPs with longer chain(n is greater than1000, crystalline form II)exhibit very low water solubility(<0.1g/100ml) (Jang et al.,2012;Laoutid et al.,2009).The APPs are stable and nonvolatile compounds.Those with long chains start decomposing at temperatures above300◦C giv-ing polyphosphoric acid and ammonia,whereas the short chain ones decompose at150◦C.It is thus important to adapt a crys-talline form of APP to the decomposition temperature of a polymer. When an APP is incorporated into a polymer that contains oxygen and/or nitrogen atoms,polymer charring occurs.Thermal degrada-tion of APP creates free acidic hydroxyl groups that condense by thermal dehydration yielding a crosslinked structure of ultraphos-phate and polyphosphoric acid with a highly crosslinked structure. Polyphosphoric acid reacts with oxygen and/or nitrogen containing polymers and catalyses their dehydration reaction and char for-mation.However,the effectiveness of an APP is dependent on the loading concentration.Low concentrations of APP are not efficient in aliphatic polyamides,but at high concentrations it becomes effi-cient.In non self-charring polymeric materials,the APP can modify the degradation mechanism of the polymer(Bourbigot&Fontaine, 2010;Ke et al.,2010;Zhu et al.,2011).2.2.1.3.Red phosphorus.This is the most concentrated source of phosphorus forflame retardancy and is used in small quantities (i.e.<10%).It is effective in oxygen and nitrogen containing poly-mers(i.e.polyesters,polyamides and polyurethanes).For oxygen containing polymers only,the mode of action involves specific scav-enging of oxygen containing radicals leading to the generation of gaseous fuel species.For oxygen and nitrogen containing polymers, red phosphorus turns into phosphoric acid or phosphoric anhy-dride,which gives polyphosphoric acid upon heating.This happens through thermal oxidation and the formed polyphosphoric acid catalyses the dehydration reaction of the polymer chain ends and triggers char formation(Laoutid et al.,2009;Laoutid,Ferry,Lopez-Cuesta,&Crespy,2006).Additionally,red phosphorus is also effective in non oxygenated polymers(e.g.polyethylene).Consequently,red phosphorus depolymerizes into white phosphorus(P4).This white phosphorus can volatilize at high temperatures and act in the gaseous phase or it can diffuse from the bulk of the polymer to the burning sur-face where it oxidizes to phosphoric acid derivatives.These can come into close contact with theflame and form phosphoric acid. This acid can act as a char forming agent and therefore physically limiting oxygen access and fuel volatilization(Laoutid et al.,2009).Red phosphorus is active in both the gas and condensed phase in polyethylene.In the gas phase,the produced PO•radicals quench the free radical process.In the condensed phase,red phosphorus lowers the heat of oxidation and also traps the free radicals.This results in improved thermal stability leading to a decrease in fuel production during burning of a material(Laoutid et al.,2009).The disadvantage of red phosphorus is that it releases toxic phosphine(PH3)through reaction with moisture due to its poor thermal stability.However,phosphine formation can be avoided by prior encapsulation of red phosphorus to improve its effective-ness as aflame retardant.Alternatively,phosphine formed at high temperatures can be trapped by taking advantage of its capacity to react with metallic salts(i.e.AgNO3,HgCl2,MoS2,HgO,PbO2,CuO, FeCl3·H2O)(Laoutid et al.,2009).2.2.1.4.Intumescentflame retardant system.Intumescentflame retardant systems were initially developed to protect fabrics,wood and coatings for metallic structures fromfire.Intumescent mate-rials are classed into thick or thinfilm intumescent coatings.The thickfilms are usually based on epoxy resins,contain agents that intumesce when exposed to heat and are available as solvent free systems.Thinfilms are available as solvent or water based sys-tems,and are applied by spray or brush roller in thinfilm coats. An intumescent system is based on the formation of an expanded carbonized layer on the surface of a polymer during thermal degra-dation.This layer acts as an insulating barrier by reducing heat transfer between the heat source and the polymer surface,by limiting the fuel transfer from the polymer towards theflame,and limiting the oxygen diffusion into a material.The formulation of an intumescent system consists of three components:an acid source,a carbonizing agent and a blowing agent.Table1tabulates examples of each component category(Bourbigot&Duquesne,2007).The intumescent FRs are widely used due to their advantages of low smoke and low toxicity(Jimenez,Duquesne,&Bourbigot,2006;Ke et al.,2010;Laoutid et al.,2009;Morgan&Gilman,2013).An acid source promotes dehydration of the carbonizing agent and results in the formation of a carbonaceous layer.It has to be liberated at a temperature below the decomposition temper-ature of a carbonizing agent and its dehydration should happen around the decomposition temperature of a polymer.A carboniz-ing agent is generally a carbohydrate that can be dehydrated by an acid to form a char.Its effectiveness relates to the number of car-bon atoms and the reactive hydroxyl sites containing carbon source agent molecules.The quantity of char produced is dependent on the number of carbon atoms present.Reactive hydroxyl(OH)sites determine the rate of the dehydration reaction and thus the rate of formation of the carbonized structure.A blowing agent decomposes and releases gas leading to expansion of the polymer and forma-tion of swollen multicellular layer.The gas must be released during thermal decomposition of a carbonizing agent in order to trigger the expansion of the carbonized layer(Bourbigot&Duquesne,2007; Jimenez et al.,2006;Ke et al.,2010;Laoutid et al.,2009;Morgan& Gilman,2013).2.2.2.Halogen basedflame retardantsHalogenated FRs are molecules that include elements from group VII of the periodic table(F,Cl,Br and I).Their effectiveness increases in the order F<Cl<Br<I.The type of halogen dictates the effectiveness of the halogenatedflame retardant.However,fluorine (F)and iodine(I)are not used because they do not interfere with the polymer combustion process.Fluorinated compounds are more thermally stable than most polymers and do not release halogen radicals at the same temperature range or below the decomposition of the polymers.Iodine compounds are less thermally stable than most commercial polymers and therefore release halogen species during polymer processing.Bromine and chlorine can readily be released and partake in the combustion process because of their low bonding energy with carbon atoms(Chen&Wang,2010; Laoutid et al.,2009;Morgan&Gilman,2013;Troitzsch,1998).2.2.2.1.Halogenatedflame retardant additives.Halogenated FRs differ in chemical structure from aliphatic to aromatic carbon。
复合材料英语【范本模板】
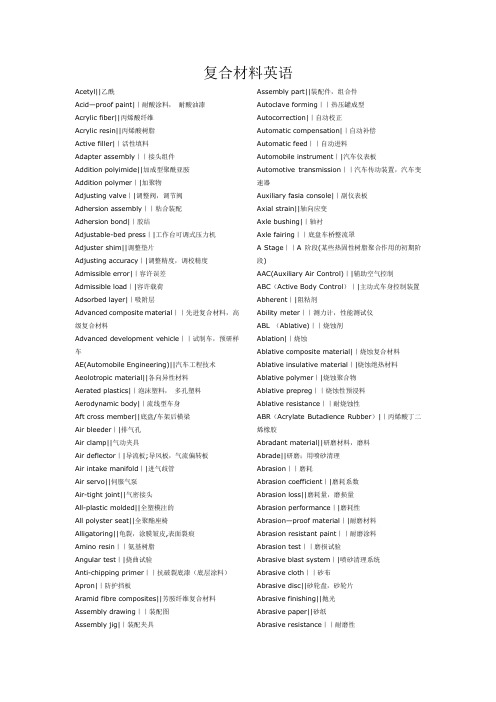
复合材料英语Acetyl||乙酰Acid—proof paint||耐酸涂料,耐酸油漆Acrylic fiber||丙烯酸纤维Acrylic resin||丙烯酸树脂Active filler||活性填料Adapter assembly||接头组件Addition polyimide||加成型聚酰亚胺Addition polymer||加聚物Adjusting valve||调整阀,调节阀Adhersion assembly||粘合装配Adhersion bond||胶结Adjustable-bed press||工作台可调式压力机Adjuster shim||调整垫片Adjusting accuracy||调整精度,调校精度Admissible error||容许误差Admissible load||容许载荷Adsorbed layer||吸附层Advanced composite material||先进复合材料,高级复合材料Advanced development vehicle||试制车,预研样车AE(Automobile Engineering)||汽车工程技术Aeolotropic material||各向异性材料Aerated plastics||泡沫塑料,多孔塑料Aerodynamic body||流线型车身Aft cross member||底盘/车架后横梁Air bleeder||排气孔Air clamp||气动夹具Air deflector||导流板;导风板,气流偏转板Air intake manifold||进气歧管Air servo||伺服气泵Air-tight joint||气密接头All-plastic molded||全塑模注的All polyster seat||全聚酯座椅Alligatoring||龟裂,涂膜皱皮,表面裂痕Amino resin||氨基树脂Angular test||挠曲试验Anti-chipping primer||抗破裂底漆(底层涂料)Apron||防护挡板Aramid fibre composites||芳胺纤维复合材料Assembly drawing||装配图Assembly jig||装配夹具Assembly part||装配件,组合件Autoclave forming||热压罐成型Autocorrection||自动校正Automatic compensation||自动补偿Automatic feed||自动进料Automobile instrument||汽车仪表板Automotive transmission||汽车传动装置,汽车变速器Auxiliary fasia console||副仪表板Axial strain||轴向应变Axle bushing||轴衬Axle fairing||底盘车桥整流罩A Stage||A 阶段(某些热固性树脂聚合作用的初期阶段)AAC(Auxiliary Air Control)||辅助空气控制ABC(Active Body Control)||主动式车身控制装置Abherent||阻粘剂Ability meter||测力计,性能测试仪ABL (Ablative)||烧蚀剂Ablation||烧蚀Ablative composite material||烧蚀复合材料Ablative insulative material||烧蚀绝热材料Ablative polymer||烧蚀聚合物Ablative prepreg||烧蚀性预浸料Ablative resistance||耐烧蚀性ABR(Acrylate Butadience Rubber)||丙烯酸丁二烯橡胶Abradant material||研磨材料,磨料Abrade||研磨;用喷砂清理Abrasion||磨耗Abrasion coefficient||磨耗系数Abrasion loss||磨耗量,磨损量Abrasion performance||磨耗性Abrasion—proof material||耐磨材料Abrasion resistant paint||耐磨涂料Abrasion test||磨损试验Abrasive blast system||喷砂清理系统Abrasive cloth||砂布Abrasive disc||砂轮盘,砂轮片Abrasive finishing||抛光Abrasive paper||砂纸Abrasive resistance||耐磨性ABS(Acrylonitrile Butadiene Styrene)resin||ABS 树脂,丙烯腈-丁二烯-苯乙烯(热塑性)树脂ABSM(American Bureau of Standard Materials)||美国标准材料局Absolute dynamic modulus||绝对动态模量Absolute error||绝对误差Absorbent material||吸收性材料,吸收性物质,吸声材料,吸收剂Absorber||减振器,阻尼器,缓冲器ACA(Automotive Composite Alliance)||汽车复合材料协会ACC(Automatic Clutch Control)||自动离合器操纵控制Accelerant||促进剂,加速剂Accelerated aging test||加速老化试验,人工老化试验Accelerator pedal shaft||加速踏板轴Accelerator pump nozzle||加速泵喷嘴Acceptable life||有效使用寿命Acceptance test specification||验收测试规范Access panel||罩板,盖板Accessory||配件,附属品Accessory equipment||辅助设备Accessory kit||附件包,成套附件Accumulator can||储电池外壳Accumulator package||蓄压器组件,蓄压器单元Accuracy in calibration||校准精度Accuracy of finish||最终加工精度Accuracy of manufacture||制造精度Accuracy of positioning||定位精度Accuracy of repetition||重现精度,复制精度Acetal matrix composites||缩醛树脂基复合材料Acetal plastic||缩醛塑料,聚甲醛塑料Acetal resin||缩醛树脂Acetamide||乙酰胺Acetate fiber||醋酸纤维,乙酸纤维Acetone||丙酮Back corner panel||后围角板Back panel||后围板Back side panel||后侧板Back wall pillar||后围立柱Backer||衬料Baffler||挡板,阻尼器;导流叶片Bag Molding||气囊施压成型(袋模法) Baggage holder||行李架Barrier coat||阻挡层;防渗涂层Batch mixing||分批混合,批混Batching unit||分批加料装置Bearing assembly||轴承组合件Biaxial winding||双角缠绕,双轴缠绕Binder fiber||粘合纤维Bipolymer||二元共聚物Bismaleimide composites||双马来酰亚胺复合材料Blank placement||坯料的放置Blanket||玻璃纤维毡;坯料Blanking press||冲压机,冲割压力机Blending resin||掺合树脂BMC(Bulk Moulding Compound)||团状膜塑料BMI (Bismaleimide)||双马来酰亚胺Body back panel||车身后板Body back wall||车身驾驶室后围Body bracket||车身支架Body control module||车身控制模块Body frame (Body skeleton)||车身骨架Body front panel||车身驾驶室前围板Body monocoque||单壳体车身,单壳式结构车身Body outer panel||驾驶室覆盖件;驾驶室覆盖件Body structural member||车身结构件Body trim||车身装饰件Bonded riveted structure||胶铆结构Bonnet||发动机罩Brake||制动器Brake arrangement||制动装置Brinell hardness test||布氏硬度试验Brittle coating||脆性涂层Bulk coat||整体涂层Bulk heat treatment||整体热处理Bulk moulding compound||(增强塑料)预制整体模塑料Bumper bracket(holder)||保险杠托架Bus brake system||客车制动系Butt flange||对接法兰Butt joint||对接接头;对接Butterfly valve||节流阀,节气门BWI (Body In White)||白车身Cab deflector shield||驾驶室导流板Cab fairing||驾驶室整流罩Cab floor||驾驶室地板Cab mounting||驾驶室悬置CAD(Computer Aided Design)||计算机辅助设计CAE (Computer Aided Engineering)||计算机辅助工程设计Calibration tolerance||校准公差Calibrating instrument||校准仪表Camouflage paint||覆面漆, 盖面涂料,伪假漆Cantilever beam impact test||悬臂梁冲击试验Carbon-felt reinforced carbon composites||碳毡增强碳复合材料Carbon fiber clutch||碳纤维离合器Carbon filament cloth||碳丝织物Case extension||外壳的伸出部分,延伸外壳Casing gasket||外壳密封垫Catalyst manifold||固化剂总成Catalyst pump||固化剂泵Catalyst ratio||固化剂比率Cavity||模槽,型腔;凹模Cavity block||阴模Cavity depth||模槽深度Cellular board||蜂窝状板,多孔板Cellular plastics||泡沫塑料,多孔塑料Centre boss||轮毂Centre pin||销轴,枢轴,主销Centrifugal casting moulding||离心浇铸成型Centrosymmetry||中心对称层板Ceramic matrix composites||陶瓷基复合材料Charge||填充气体,填充料Chasis||底盘;机壳,车架Chlorinated polyethlene||聚氯乙烯Chopped fiber||短切纤维Chopped random mat||短切无序毡Chopped strand||短切原丝CIRTM(Co—Injection RTM)||共注射RTM Clamping fixture||夹具,夹紧装置Clamping force||夹持力,合模力Class A surface||A级表面Clear coat||透明涂层,透明罩漆,清漆层Clear coat finish||清漆涂层Clicker die||冲模Climb milling||同向铣削,顺铣Clipping press||切边压力机Closure pressing speed||合模速度CMM(Closed Mould Moulding)||闭合模塑CMT(Compression Molding||挤压成型工艺CNC(Computerized Numerical Control)||电脑数值控制Coarse grinding||粗磨,用砂轮初加工Coating defect||涂层缺陷Collision test||碰撞试验,撞车试验Combination property||综合性能Concept design||概念设计Convection modulus||对流模量Convergence test||收敛试验Cooling fixture||冷却夹具Cooling tower||冷却塔Crazing||龟裂,细裂纹Cresol resin||甲酚树脂Cutting felt||毡的剪切Cutting—off bushing||环形下料模; 下料环Damped structure||阻尼缓冲结构Damper bracket||件振器支架Dashboard illumination||仪表板照明Dash trimming||前围板衬板Deburring||去毛刺,倒角,除飞边Deepdrawing forming||深拉成型Deflection test||挠曲试验Dent resistance||耐冲击性Design freedom||设计自由度Detail drawing||祥图,零件图Die assembly||压模装置Die casting||压模铸件,压模铸法Dimethyl fomamide||二甲基甲酰胺Dimethyl ketone||二甲基甲酮; 丙酮Dip pretreatment||浸渍预处理Die prime coat||浸渍打底漆Dimensional stability||尺寸稳定性Dip coating||浸涂Dip forming||浸渍成型Durability testing||耐久性试验,寿命试验Dwell||保压,暂停加压;滞留时间Dynamometer||测力计Edge effect||边缘效应,边界效应Edge feed||边缘进料Edge gate||侧浇口Ejection force||脱模力Ejector||起模杆Ejector guide pillar||推板导套Ejector housing||支架Elasticizer||增塑剂Elastomeric composites||高弹体复合材料Elongation at break||断裂延伸率Energy absorbing foam||吸能泡沫塑料Epoxy resin||环氧树脂Ether ketone||酮醚Explosion proof||防爆Exterior body panelling||车身外板部蒙皮Exterior trim||外饰,外饰件Fabric composites||织物复合材料Fabric impregnation||织物浸渍Fabric preform||织物预成型Fabric prereg||织物预浸料Fabrication parameter||制造参数Fabrication procedure||制造工序Fabricating machinery||加工设备Face plate coupling||法兰式连接Factory primer||工厂底漆,工厂防锈漆Fairing||整流罩,整流装置Fairing panel||前裙板Fascia bracket||仪表板支架Fascia mask||仪表板罩板Fastening clamp||夹紧装置,紧固夹子Fatigue tension test||拉伸疲劳性试验FCM(Fibrous Composite material)||纤维复合材料FEA(Finite Element Anlysis)||有限元分析Feed system||供料系统Feeding pump||供给泵Feeding speed||进给速度Female groove||凹模Female mould(tooling)||阴模Fender||翼子板;护板Fender apron||挡泥板Fender inner panel||翼子板内衬护板Fiber composite laminate||纤维复合材料层板Fiber mat layer||纤维毡层Finisher(Finishing component)||装饰件Flange||法兰, 凸缘Flange fitting||法兰式管接头Flash||毛边Flash mold||毛边模具Front sheet metal||车前板制件Fuselage fairing||机身整流装置Gage kit||仪表组,仪表套件Gas cavity||气泡,砂眼Gauge panel||仪表板Gear assembly||齿轮传动装置, 减速器Gearbox cover||变速器壳盖Gear bracket support||齿轮托支架Gel coat||胶衣,凝胶涂层Gel coat drum||胶衣圆桶Gel coat flow monitor||胶衣流量监控器Gel time||凝胶时间Glass fiber winding machine||玻璃纤维缠绕机Glass wool||玻璃棉Glass yarn||玻璃丝Guiding device||导向装置Gunk||预混料Gusset||角撑件Gutter channel||流水槽Hand lay-up ||手工铺叠,手工铺贴Hardness testing machine||硬度测试仪Hauling truck||拖车Header board outside panel||前板外板Headrest||靠枕Heat barrier material||隔热材料Heat forming||热成型High molecular material||高分子材料High pressure bag molding||高压袋成型工艺High pressure injection moulding||高压注射成型,高压注射模塑High—strength structural adhesives||高强度结构粘合剂此资源来自:由FanE『翻译中国』http;//www.FanE。
【高分子专业英语翻译】
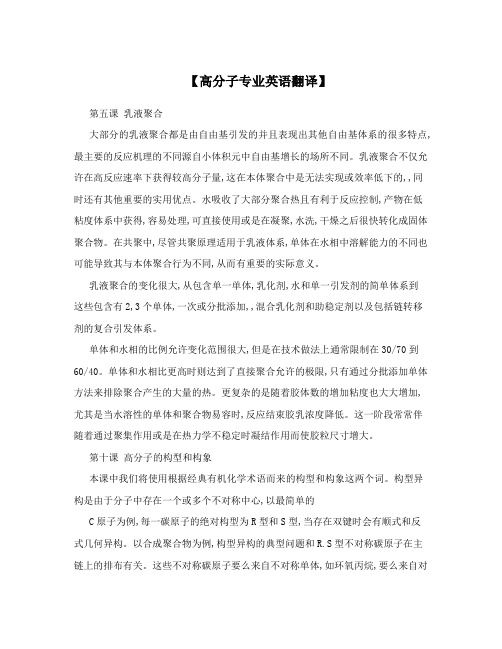
【高分子专业英语翻译】第五课乳液聚合大部分的乳液聚合都是由自由基引发的并且表现出其他自由基体系的很多特点,最主要的反应机理的不同源自小体积元中自由基增长的场所不同。
乳液聚合不仅允许在高反应速率下获得较高分子量,这在本体聚合中是无法实现或效率低下的,,同时还有其他重要的实用优点。
水吸收了大部分聚合热且有利于反应控制,产物在低粘度体系中获得,容易处理,可直接使用或是在凝聚,水洗,干燥之后很快转化成固体聚合物。
在共聚中,尽管共聚原理适用于乳液体系,单体在水相中溶解能力的不同也可能导致其与本体聚合行为不同,从而有重要的实际意义。
乳液聚合的变化很大,从包含单一单体,乳化剂,水和单一引发剂的简单体系到这些包含有2,3个单体,一次或分批添加,,混合乳化剂和助稳定剂以及包括链转移剂的复合引发体系。
单体和水相的比例允许变化范围很大,但是在技术做法上通常限制在30/70到60/40。
单体和水相比更高时则达到了直接聚合允许的极限,只有通过分批添加单体方法来排除聚合产生的大量的热。
更复杂的是随着胶体数的增加粘度也大大增加,尤其是当水溶性的单体和聚合物易容时,反应结束胶乳浓度降低。
这一阶段常常伴随着通过聚集作用或是在热力学不稳定时凝结作用而使胶粒尺寸增大。
第十课高分子的构型和构象本课中我们将使用根据经典有机化学术语而来的构型和构象这两个词。
构型异构是由于分子中存在一个或多个不对称中心,以最简单的C原子为例,每一碳原子的绝对构型为R型和S型,当存在双键时会有顺式和反式几何异构。
以合成聚合物为例,构型异构的典型问题和R.S型不对称碳原子在主链上的排布有关。
这些不对称碳原子要么来自不对称单体,如环氧丙烷,要么来自对称单体,如乙烯单体,,这些物质的聚合,在每个单体单元中形成至少一个不对称碳原子。
大分子中的构型异构源于侧链上存在不对称的碳原子,例如不对称乙烯单体的聚合,也是可能的,现今已经被广泛研究。
和经典有机化学术语一致,构象,旋转体,旋转异构体,构象异构体,指的是由于分子单键的内旋转而形成的空间排布的不同。
高分子专业英语.
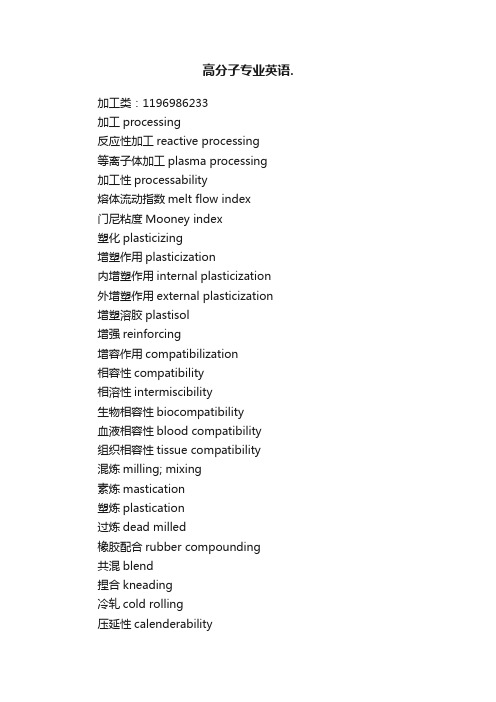
高分子专业英语.加工类:1196986233加工processing反应性加工reactive processing等离子体加工plasma processing加工性processability熔体流动指数melt flow index门尼粘度Mooney index塑化plasticizing增塑作用plasticization内增塑作用internal plasticization外增塑作用external plasticization增塑溶胶plastisol增强reinforcing增容作用compatibilization相容性compatibility相溶性intermiscibility生物相容性biocompatibility血液相容性blood compatibility组织相容性tissue compatibility混炼milling; mixing素炼mastication塑炼plastication过炼dead milled橡胶配合rubber compounding共混blend捏合kneading冷轧cold rolling压延性calenderability压延calendering埋置embedding压片preforming模塑molding模压成型compression molding压缩成型compression forming冲压模塑impact moulding, shock moulding 叠模压塑stack moulding复合成型composite molding注射成型injection molding注塑压缩成型injection compression molding射流注塑jet molding无流道冷料注塑runnerless injection molding共注塑coinjection molding气辅注塑gas aided injection molding注塑焊接injection welding传递成型transfer molding树脂传递成型resin transfer molding铸塑cast熔铸fusion casting铸塑成型casting molding单体浇铸monomer casting挤出extrusion共挤出co-extrusion多层挤塑multi-layer extrusion共挤吹塑co-extrusion blow molding同轴挤塑coaxial extrusion吹胀挤塑blown extrusion挤出吹塑extrusion blow molding挤拉吹塑成型extrusion draw blow molding反应性挤塑reactive extrusion固相挤出solid-phase extrusion发泡expanding foam后发泡post expansion物理发泡physical foam化学发泡chemical foam吹塑blow molding多层吹塑multi-layer blow molding拉伸吹塑成型stretch blow molding滚塑rotational moulding反应注射成型reaction injection molding, RIM 真空成型vacuum foaming无压成型zero pressure molding真空烧结vacuum sintering真空袋成型vacuum bag molding热成型thermal forming拉伸热成型stretch thermoforming袋模塑bag molding糊塑paste molding镶铸imbedding冲压成型impact molding触压成型impression molding层压材料laminate泡沫塑料成型foam molding包模成型drape molding充气吹胀inflation橡胶胶乳rubber latex高分子胶体polymer colloid生橡胶raw rubber, crude rubber硬质胶ebonite再生胶reclaimed rubber充油橡胶oil-extended rubber母胶masterbatch交联crosslinking固化cure光固化photo-cure硫化vulcanization后硫化post cure, post vulcanization 自硫化bin cure自交联self crosslinking过硫over cure返硫reversion欠硫under cure动态硫化dynamic vulcanization不均匀硫化heterogeneous vulcanization 开始效应set-up effect自动硫化self-curing, self-vulcanizing 焦烧scorching无压硫化non-pressure cure模压硫化moulding curing常温硫化auto-vulcanization热硫化heat curing蒸汽硫化steam curing微波硫化micro wave curing辐射硫化radiation vulcanization辐射交联radiation crosslinking连续硫化continuous vulcanization无模硫化open vulcanization成纤fiber forming可纺性spinnability纺丝spinning干纺dry spinning湿纺wet spinning干施法纺丝dry wet spinning干喷湿法纺丝dry jet wet spinning溶液纺丝solution spinning乳液闪蒸纺丝法emulsion spinning喷射纺丝jet spinning喷纺成型spray spinning液晶纺丝liquid crystal spinning熔纺melt spinning共混纺丝blended spinning凝胶纺丝gel spinning熔纺melt spinning共混纺丝blended spinning凝胶纺丝gel spinning反应纺丝reaction spinning静电纺丝electrostatic spinning高压纺丝high-pressure spinning复合纺丝conjugate spinning无纺布non-woven fabrics单丝monofilament, monofil复丝multifilament全取向丝fully oriented yarn中空纤维hollow fiber皮芯纤维sheath core fiber共纺co-spinning冷拉伸cold drawing, cold stretching单轴拉伸uniaxial drawing, uniaxial elongation 双轴拉伸biaxial drawing多轴拉伸multiaxial drawing皮心效应skin and core effect皮层效应skin effect防缩non-shrink熟成ripening垂挂sag定型sizing起球现象pilling effect捻度twist旦denier特tex纱yarn股strand粘合adhesion反应粘合reaction bonding压敏粘合pressure sensitive adhesion底漆primer浸渍impregnation浸渍树脂solvent impregnated resin基体matrix聚合物表面活性剂polymeric surfactant高分子絮凝剂polymeric flocculant预发颗粒pre-expanded高分子模polymeric membraneH-模H-filmLB膜Langmuir Blodgett film(LB film)半透膜semipermeable membrane反渗透膜reverse osmosis membrane多孔膜porous membrane各向异性膜anisotropic membrane正离子交换膜cationic exchange membrane 负离子交换膜anionic exchange membrane吸附树脂polymeric adsorbent添加剂additive固化剂curing agent潜固化剂latent curing agent硫化剂vulcanization agent给硫剂sulfur donor agent, sulfur donor 硫化促进剂vulcanization accelerator硫化活化剂vulcanization activator活化促进剂activating accelerator活化剂activator防焦剂scorch retarder抗硫化返原剂anti-reversion agent塑解剂peptizer偶联剂coupling agent硅烷偶联剂silane coupling agent钛酸酯偶联剂titanate coupling agent铝酸酯偶联剂aluminate coupling agent增强剂reinforcing agent增硬剂hardening agent惰性填料inert filler增塑剂plasticizer增粘剂tackifier增容剂compatibilizer增塑增容剂plasticizer extender分散剂dispersant agent结构控制剂constitution controller色料colorant荧光增白剂optical bleaching agent抗降解剂antidegradant防老剂anti-aging agent防臭氧剂antiozonant抗龟裂剂anticracking agent抗疲劳剂anti-fatigue agent抗微生物剂biocide防蚀剂antip-corrosion agent光致抗蚀剂photoresist防霉剂antiseptic防腐剂rot resistor防潮剂moisture proof agent防臭剂re-odorant抗氧剂antioxidant抗静电添加剂antistatic additive抗静电剂antistatic agent紫外线稳定剂ultraviolet stabilizer紫外光吸收剂ultraviolet absorber光稳定剂light stabilizer, photostabilizer 光屏蔽剂light screener发泡剂foaming agent物理发泡剂physical foaming agent化学发泡剂chemical foaming agent脱模剂releasing agent内脱模剂internal releasing agent外脱模剂external releasing agent阻燃剂flame retardant防火剂fire retardant烧蚀剂ablator润滑剂lubricant湿润剂wetting agent隔离剂separant增韧剂toughening agent抗冲击改性剂impact modifier消泡剂antifoaming agent减阻剂drag reducer破乳剂demulsifier粘度改进剂viscosity modifier增稠剂thickening agent, thickener阻黏剂abhesive洗脱剂eluant附聚剂agglomerating agent后处理剂after-treating agent催干剂drier防结皮剂anti-skinning agent纺织品整理剂textile finishing agent高物高化类:结构单元constitutional unit重复结构单元constitutional repeating unit 构型单元configurational unit立构重复单元stereorepeating unit立构规整度tacticity等规度isotacticity间同度syndiotacticity无规度atacticity嵌段block规整嵌段regular block非规整嵌段irregular block立构嵌段stereoblock有规立构嵌段isotactic block无规立构嵌段atactic block单体单元monomeric unit二单元组diad三单元组triad四单元组tetrad五单元组pentad无规线团random coil自由连接链freely-jointer chain自由旋转链freely-rotating chain蠕虫状链worm-like chain柔性链flexible chain链柔性chain flexibility刚性链rigid chain链刚性chain rigidity棒状链rodlike chain聚集aggregation聚集体aggregate凝聚、聚集coalescence链缠结chain entanglement凝聚缠结cohesional entanglement物理缠结physical entanglement拓扑缠结topological entanglement凝聚相condensed phase凝聚态condensed state凝聚过程condensing process临界聚集浓度critical aggregation concentration 线团-球粒转换coil-globule transition受限链confined chain受限态confined state物理交联physical crosslinking统计线团statistical coil等效链equivalent chain统计链段statistical segment链段chain segment链构象chain conformation无规线团模型random coil model无规行走模型random walk model自避随机行走模型self avoiding walk model卷曲构象coiled conformation高斯链Gaussian chain无扰尺寸unperturbed dimension扰动尺寸perturbed dimension热力学等效球thermodynamically equivalent sphere近程分子内相互作用short-range intramolecular interaction 远程分子内相互作用long-range intramolecular interaction 链间相互作用interchain interaction链间距interchain spacing长程有序long range order近程有序short range order回转半径radius of gyration末端间矢量end-to-end vector链末端chain end末端距end-to-end distance无扰末端距unperturbed end-to-end distance均方根末端距root-mean-square end-to-end distance伸直长度contour length相关长度persistence length主链;链骨架chain backbone支链branch chain链支化chain branching短支链short-chain branch长支链long-chain branch支化系数branching index支化密度branching density支化度degree of branching交联度degree of crosslinking网络network网络密度network density溶胀swelling平衡溶胀equilibrium swelling分子组装molecular assembly自组装self assembly微凝胶microgel凝胶点gel point可逆凝胶reversible gel溶胶-凝胶转化sol-gel transformation临界胶束浓度critical micelle concentration组成非均一性constitutional heterogeneity, compositional heterogeneity 摩尔质量平均molar mass average数均分子量number-average molecular weight重均分子量weight-average molecular weightZ-均分子量Z-average molecular weight黏均分子量viscosity-average molecular表观摩尔质量apparent molar mass表观分子量apparent molecular weight聚合度degree of polymerization动力学链长kinetic chain length单分散性monodispersity临界分子量critical molecular weight分子量分布molecular weight distribution多分散性指数polydispersity index平均聚合度average degree of polymerization质量分布函数mass distribution function数量分布函数number distribution function重量分布函数weight distribution function舒尔茨-齐姆分布Schulz-zimm distribution最概然分布most probable distribution对数正态分布logarithmic normal distribution聚合物溶液polymer solution聚合物-溶剂相互作用polymer-solvent interaction溶剂热力学性质thermodynamic quality of solvent均方末端距mean square end to end distance均方旋转半径mean square radius of gyration良溶剂good solvent不良溶剂poor solvent位力系数Virial coefficient排除体积excluded volume溶胀因子expansion factor溶胀度degree of swelling弗洛里-哈金斯理论Flory-Huggins theory哈金斯公式Huggins equation哈金斯系数Huggins coefficient溶度参数solubility parameter摩擦系数frictional coefficient流体力学体积hydrodynamically equivalent sphere珠-棒模型bead-rod model棒-簧链模型ball-spring model流动双折射flow birefringence, streaming birefringence 动态光散射dynamic light scattering小角激光光散射low angle laser light scattering沉降平衡sedimentation equilibrium沉降系数sedimentation coefficient沉降速度法sedimentation velocity method沉降平衡法sedimentation equilibrium method相对黏度relative viscosity相对黏度增量relative viscosity黏度比viscosity ratio黏数viscosity number[乌式]稀释黏度计[Ubbelohde] dilution viscometer毛细管黏度计capillary viscometer落球黏度计ball viscometer落球黏度ball viscosity本体黏度bulk viscosity比浓黏度reduced viscosity比浓对数黏度inherent viscosity, logarithmic viscosity number 特性黏数intrinsic viscosity, limiting viscosity number黏度函数viscosity function零切变速率黏度zero shear viscosity端基分析analysis of end group蒸气压渗透法vapor pressure osmometry, VPO辐射的相干弹性散射coherent elastic scattering of radiation折光指数增量refractive index increment瑞利比Rayleigh ratio超瑞利比excess Rayleigh ratio粒子散射函数particle scattering function粒子散射因子particle scattering factor齐姆图Zimm plot散射的非对称性dissymmetry of scattering解偏振作用depolarization分级fractionation沉淀分级precipitation fractionation萃取分级extraction fractionation色谱分级chromatographic fractionation柱分级column fractionation洗脱分级elution fractionation热分级thermal fractionation凝胶色谱法gel chromatography摩尔质量排除极限molar mass exclusion limit溶剂梯度洗脱色谱法solvent gradient elution chromatography 分子量排除极限molecular weight exclusion limit洗脱体积elution volume普适标定universal calibration加宽函数spreading function链轴chain axis等同周期identity period链重复距离chain repeating distance晶体折叠周期crystalline fold period构象重复单元conformational repeating unit几何等效geometrical equivalence螺旋链helix chain构型无序configurational disorder链取向无序chain orientational disorder构象无序conformational disorder锯齿链zigzag chain双螺旋double stranded helix分子链大尺度取向global chain orientation结晶聚合物crystalline polymer半结晶聚合物semi-crystalline高分子晶体polymer crystal高分子微晶polymer crystallite结晶度degree of crystallinity高分子同晶现象macromolecular isomorphosm 聚合物形态学morphology of polymer片晶lamella, lamellar crystal轴晶axialite树枝晶体dendrite纤维晶fibrous crystal串晶结构shish-kebab structure球晶spherulite折叠链folded chain链折叠chain folding折叠表面fold surface折叠面fold plane折叠微区fold domain相邻再入模型adjacent re-entry model插线板模型switchboard model缨状微束模型fringed-micelle model折叠链晶体folded-chain crystal平行链晶体parallel-chain crystal伸展链晶体extended-chain crystal球状链晶体globular-chain crystal长周期long period近程结构short-range structure远程结构long-range structure成核作用nucleation分子成核作用molecular nucleation阿夫拉米方程Avrami equation主结晶primary crystallization后期结晶secondary crystallization外延结晶,附生结晶epitaxial crystallization 外延晶体生长,附生晶体生长epitaxial growth 织构texture液晶态liquid crystal state溶致性液晶lyotopic liquid crystal热致性液晶thermotropic liquid crystal热致性介晶thermotropic mesomorphism近晶相液晶smectic liquid crystal近晶中介相smectic mesophase近晶相smectic phase条带织构banded texture环带球晶ringed spherulite向列相nematic phase盘状相discotic phase解取向disorientation分聚segregation非晶相amorphous orientation非晶区amorphous orientation非晶态amorphous state非晶取向amorphous orientation链段运动segmental motion亚稳态metastable state相分离phase separation亚稳相分离spinodal decomposition微相miscibility界面相boundary phase相容性compatibility混溶性miscibility不相容性incompatibility不混溶性immiscibility增容作用compatibilization最低临界共溶温度lower critical solution temperature 最高临界共溶温度upper critical solution temperature 浓度猝灭concentration quenching激基缔合物荧光excimer fluorescence激基复合物荧光exciplex fluorescence激光共聚焦荧光显微镜laser confocal fluorescence microscopy 单轴取向uniaxial orientation双轴取向biaxial orientation, biorientation取向度degree of orientation橡胶态rubber state玻璃态glassy state高弹态elastomeric state黏流态viscous flow state伸长elongation高弹形变high elastic deformation回缩性nerviness拉伸比draw ratio, extension ratio泊松比Poisson’ ratio杨氏模量Young’s modulus本体模量bulk modulus剪切模量shear modulus法向应力normal stress剪切应力shear stress剪切应变shear strain屈服yielding颈缩现象necking屈服应力yield stress屈服应变yield strain脆性断裂brittle fracture脆性开裂brittle cracking脆-韧转变brittle ductile transition脆化温度brittleness temperature延性破裂ductile fracture冲击强度impact strength拉伸强度tensile strength极限拉伸强度ultimate tensile strength抗撕强度tearing strength弯曲强度flexural strength, bending strength 弯曲模量bending modulus弯曲应变bending strain弯曲应力bending stress收缩开裂shrinkage crack剪切强度shear strength剥离强度peeling strength疲劳强度fatigue strength挠曲deflection压缩强度compressive strength压缩永久变形compressive set压缩变形compressive deformation压痕硬度indentation hardness洛氏硬度Rockwell hardness布氏硬度Brinell hardness抗刮性scrath resistance断裂力学fracture mechanics力学破坏mechanical failure应力强度因子stress intensity factor断裂伸长elongation at break屈服强度yield strength断裂韧性fracture toughness弹性形变elastic deformation弹性滞后elastic hysteresis弹性elasticity弹性模量modulus of elasticity弹性回复elastic recovery不可回复形变irrecoverable deformation 裂缝crack 银纹craze形变;变形deformation永久变形deformation set剩余变形residual deformation剩余伸长residual stretch回弹,回弹性resilience延迟形变retarded deformation延迟弹性retarded elasticity可逆形变reversible deformation应力开裂stress cracking应力-应变曲线stress strain curve拉伸应变stretch strain拉伸应力弛豫tensile stress relaxation热历史thermal history热收缩thermoshrinking牛辫分析torsional braid analysis应力致白stress whitening应变能strain energy应变张量strain tensor剩余应力residual stress应变硬化strain hardening应变软化strain softening电流变液electrorheological fluid假塑性pseudoplastic拉胀性auxiticity牛顿流体Newtonian fluid非牛顿流体non-Newtonian fluid宾汉流体Bingham fluid冷流cold flow牛顿剪切粘度Newtonian shear viscosity 剪切粘度shear viscosity表观剪切粘度apparent shear viscosity 剪切变稀shear thinning 触变性thixotropy塑性形变plastic deformation塑性流动plastic flow体积弛豫volume relaxation拉伸黏度extensional viscosity粘弹性viscoelasticity线性粘弹性linear viscoelasticity非线性粘弹性non-linear viscoelasticity蠕变creep弛豫relaxation弛豫模量relaxation modulus蠕变柔量creep compliance热畸变温度heat distortion temperature弛豫谱relaxation spectrum推迟时间谱retardation spectrum弛豫时间relaxation time推迟时间retardation time动态力学行为dynamic mechanical behavior动态粘弹性dynamic viscoelasticity热-机械曲线thermo-mechanical curve动态转变dynamic transition储能模量storage modulus损耗模量loss modulus复数模量complex modulus复数柔量complex compliance动态黏度dynamic viscosity复数黏度complex viscosity复数介电常数complex dielectric permittivity介电损耗因子dielectric dissipation factor介电损耗常数dielectric loss constant介电弛豫时间dielectric relaxation time玻璃化转变glass transition玻璃化转变温度glass transition temperature次级弛豫secondary relaxation次级转变secondary transition次级弛豫温度secondary relaxation temperature开尔文模型Kelvin model麦克斯韦模型Maxwell model时-温叠加原理time-temperature superposition principle 波尔兹曼叠加原理Boltzmann superposition principle平衡因子shift factorWLF公式WLF[Williams-Lendel-Ferry] equation 软化温度softening temperature平衡熔点equilibrium melting point物理老化physical aging光老化photo-aging热老化thermal aging热氧老化thermo-oxidative aging人工老化artificial aging加速老化accelerated aging计算机模拟computer simulation分子动力学模拟molecular dynamics simulation蒙特卡洛模拟Monte Carlo simulation聚合反应类单体monomer官能度functionality平均官能度average functionality双官能单体bifunctional monomer三官能单体trifunctional monomer乙烯基单体vinyl monomer1,1-亚乙烯基单体/偏[二]取代乙烯单体vinylidene monomer 1,2-亚乙烯基单体/1,2-二取代乙烯单体vinylene monomer 双烯单体diene monomer极性单体polar monomer非极性单体non polar monomer共轭单体conjugated monomer非共轭单体non conjugated monomer活化单体activated monomer官能单体functional monomer大分子单体macromonomer环状单体cyclic monomer共聚单体co-monomer聚合反应polymerization均聚反应homopolymerization低聚反应/齐聚反应oligomerization调聚反应telomerization自发聚合spontaneous polymerization预聚合prepolymerization后聚合post polymerization再聚合repolymerization铸塑聚合cast polymerization链式聚合chain polymerization烯类聚合,乙烯基聚合vinyl polymerization双烯聚合diene polymerization加成聚合addition polymerization自由基聚合free radical polymerization可控自由基聚合controlled radical polymerization活性自由基聚合living radical polymerization原子转移自由基聚合atom transfer radical polymerization反向原子转移自由基聚合reverse atom transfer radical polymerization可逆加成断裂链转移reversible addition fragmentation chain transfer氮氧调控聚合nitroxide mediated polymerization稳定自由基聚合stable free radical polymerization自由基异构化聚合free radical isomerization polymerization 自由基开环聚合radical ring opening polymerization氧化还原聚合redox polymerization无活性端聚合,死端聚合dead end polymerization光致聚合photo polymerization光引发聚合light initiated polymerization光敏聚合photosensitized polymerization四中心聚合four center polymerization电荷转移聚合charge transfer polymerization辐射引发聚合radiation initiated polymerization热聚合thermal polymerization电解聚合electrolytic polymerization等离子聚合plasma polymerization易位聚合metathesis polymerization开环易位聚合ring opening metathesis polymerization精密聚合precision polymerization环化聚合cyclopolymerization拓扑化学聚合topochemical polymerization平衡聚合equilibrium polymerization离子聚合ionic polymerization辐射离子聚合radiation ion polymerization离子对聚合ion pair polymerization正离子聚合/阳离子聚合cationic polymerization碳正离子聚合carbenium ion polymerization, carbocationic polymerization 假正离子聚合pseudo cationic polymerization 假正离子活性聚合pseudo cationic living polymerization活性正离子聚合living cationic polymerization负离子聚合,阴离子聚合anionic polymerization碳负离子聚合carbanionic polymerization活性负离子聚合living anionic polymerization负离子环化聚合anionic cyclopolymerization负离子电化学聚合anionic electrochemical polymerization负离子异构化聚合anionic isomerization polymerization烯丙基聚合allylic polymerization活性聚合living polymerization两性离子聚合zwitterion polymerization齐格勒-纳塔聚合Ziegler Natta polymerization配位聚合coordination polymerization配位离子聚合coordinated ionic polymerization配位负离子聚合coordinated anionic polymerization配位正离子聚合coordinated cationic polymerization插入聚合insertion polymerization定向聚合,立构规整聚合stereoregular polymerization, stereospecific polymerization 有规立构聚合tactic polymerization 全同立构聚合isospecific polymerization不对称诱导聚合asymmetric induction polymerization不对称选择性聚合asymmetric selective polymerization不对称立体选择性聚合asymmetric stereoselective polymerization对映体不对称聚合enantioasymmetric polymerization对映体对称聚合enantiosymmetric polymerization异构化聚合isomerization polymerization氢转移聚合hydrogen transfer polymerization基团转移聚合group transfer polymerization消除聚合elimination polymerization模板聚合matrix polymerization, template polymerization 插层聚合intercalation polymerization无催化聚合uncatalyzed polymerization开环聚合ring opening polymerization活性开环聚合living ring opening polymerization不死的聚合immortal polymerization酶聚合作用enzymatic polymerization聚合加成反应,逐步加成聚合polyaddition偶联聚合coupling polymerization序列聚合sequential polymerization闪发聚合,俗称暴聚flash polymerization氧化聚合oxidative polymerization氧化偶联聚合oxidative coupling polymerization逐步聚合step growth polymerization缩聚反应condensation polymerization, polycondensation 酯交换聚合transesterification type polymerization自催化缩聚autocatalytic polycondensation均相聚合homogeneous polymerization非均相聚合heterogeneous polymerization相转化聚合phase inversion polymerization本体聚合bulk polymerization, mass polymerization固相聚合solid phase polymerization气相聚合gaseous polymerization, gas phase polymerization 吸附聚合adsorption polymerization溶液聚合solution polymerization沉淀聚合precipitation polymerization淤浆聚合slurry polymerization悬浮聚合suspension polymerization反相悬浮聚合reversed phase suspension polymerization 珠状聚合bead polymerization, pearl polymerization分散聚合dispersion polymerization。
GBT 224-2019《钢的脱碳层深度测定法》中金相法之缺陷
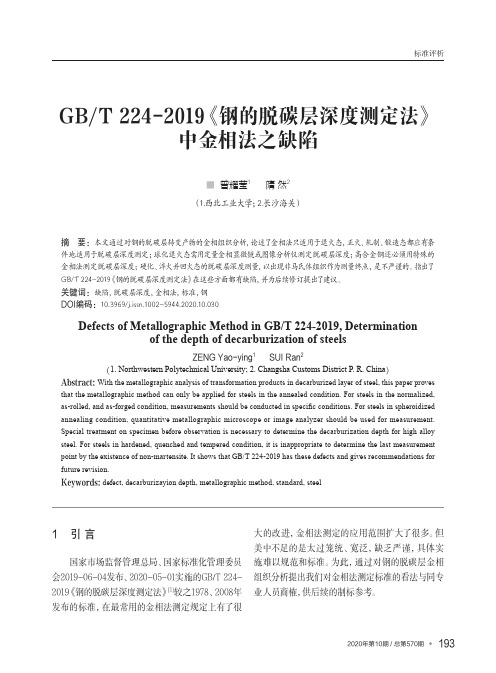
GB/T 224-2019《钢的脱碳层深度测定法》中金相法之缺陷■ 曾耀莹1 隋 然2(1.西北工业大学;2.长沙海关)摘 要:本文通过对钢的脱碳层转变产物的金相组织分析,论述了金相法只适用于退火态,正火、轧制、锻造态都应有条件地适用于脱碳层深度测定;球化退火态需用定量金相显微镜或图像分析仪测定脱碳层深度;高合金钢还必须用特殊的金相法测定脱碳层深度;硬化、淬火并回火态的脱碳层深度测量,以出现非马氏体组织作为测量终点,是不严谨的。
指出了GB/T 224-2019《钢的脱碳层深度测定法》在这些方面都有缺陷,并为后续修订提出了建议。
关键词:缺陷,脱碳层深度,金相法,标准,钢DOI编码:10.3969/j.issn.1002-5944.2020.10.030Defects of Metallographic Method in GB/T 224-2019, Determinationof the depth of decarburization of steelsZENG Yao-ying1 SUI Ran2(1. Northwestern Polytechnical University; 2. Changsha Customs District P. R. China)Abstract: With the metallographic analysis of transformation products in decarburized layer of steel, this paper proves that the metallographic method can only be applied for steels in the annealed condition. For steels in the normalized, as-rolled, and as-forged condition, measurements should be conducted in specific conditions. For steels in spheroidized annealing condition, quantitative metallographic microscope or image analyzer should be used for measurement. Special treatment on specimen before observation is necessary to determine the decarburization depth for high alloy steel. For steels in hardened, quenched and tempered condition, it is inappropriate to determine the last measurement point by the existence of non-martensite. It shows that GB/T 224-2019 has these defects and gives recommendations for future revision.Keywords: defect, decarburizayion depth, metallographic method, standard, steel标准评析1 引 言国家市场监督管理总局、国家标准化管理委员会2019-06-04发布、2020-05-01实施的GB/T 224-2019《钢的脱碳层深度测定法》[1]较之1978、2008年发布的标准,在最常用的金相法测定规定上有了很大的改进,金相法测定的应用范围扩大了很多。
生物降解塑料中英文对照
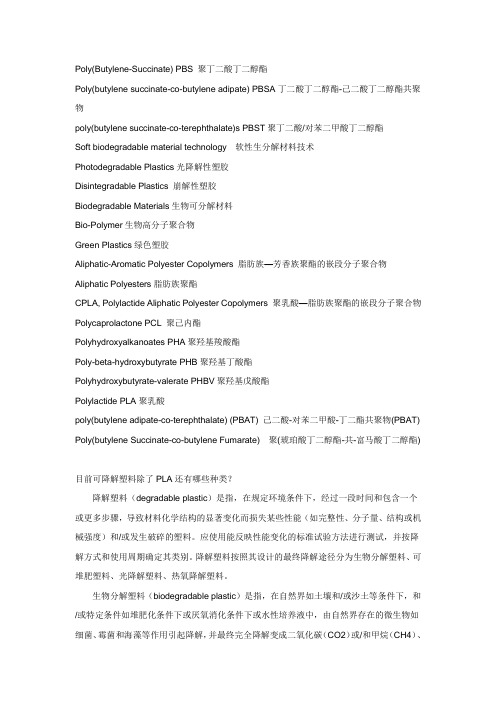
Poly(Butylene-Succinate) PBS 聚丁二酸丁二醇酯Poly(butylene succinate-co-butylene adipate) PBSA丁二酸丁二醇酯-己二酸丁二醇酯共聚物poly(butylene succinate-co-terephthalate)s PBST聚丁二酸/对苯二甲酸丁二醇酯Soft biodegradable material technology 软性生分解材料技术Photodegradable Plastics光降解性塑胶Disintegradable Plastics 崩解性塑胶Biodegradable Materials生物可分解材料Bio-Polymer生物高分子聚合物Green Plastics绿色塑胶Aliphatic-Aromatic Polyester Copolymers 脂肪族—芳香族聚酯的嵌段分子聚合物Aliphatic Polyesters脂肪族聚酯CPLA, Polylactide Aliphatic Polyester Copolymers 聚乳酸—脂肪族聚酯的嵌段分子聚合物Polycaprolactone PCL 聚己内酯Polyhydroxyalkanoates PHA聚羟基羧酸酯Poly-beta-hydroxybutyrate PHB聚羟基丁酸酯Polyhydroxybutyrate-valerate PHBV聚羟基戊酸酯Polylactide PLA聚乳酸poly(butylene adipate-co-terephthalate) (PBAT) 己二酸-对苯二甲酸-丁二酯共聚物(PBAT) Poly(butylene Succinate-co-butylene Fumarate) 聚(琥珀酸丁二醇酯-共-富马酸丁二醇酯)目前可降解塑料除了PLA还有哪些种类?降解塑料(degradable plastic)是指,在规定环境条件下,经过一段时间和包含一个或更多步骤,导致材料化学结构的显著变化而损失某些性能(如完整性、分子量、结构或机械强度)和/或发生破碎的塑料。
Large Deformation Plasticity of Amorphous Solids, with 非晶态固体大变形塑性,和

To be objective (in general): g* Qg
G* QGQT
F QF Fe QFe Le QLeQ TQ Q T
The relaxed and reference configurations are invariant to the transformations of the Eulerian Space
y (X ,t) y * (X ,t) Q ( t)y (X ,t) q ( t)
Principle of Relativity: relation of the two motions is equivalent Q(t) Relative motion of two observers Eulerian bases
Experimental Results – Polycarbonate
From Boyce and Arruda
COMPRESSION
TENSION
• Large deformation regime • Strain-softening after initial yield • Back stress evolution after yield drop to create strain-hardening
Motivation – Examples of Materials
• Amorphous Solids –
– polymeric and metallic glasses (i.e. Polycarbonate) – Rubber degradation
• Biomaterials
– Soft Collageneous Biological Tissue (i.e. cartilage, cervical tissue, skin, tendon, etc.)
阻燃降解环氧树脂的研究现状的英文
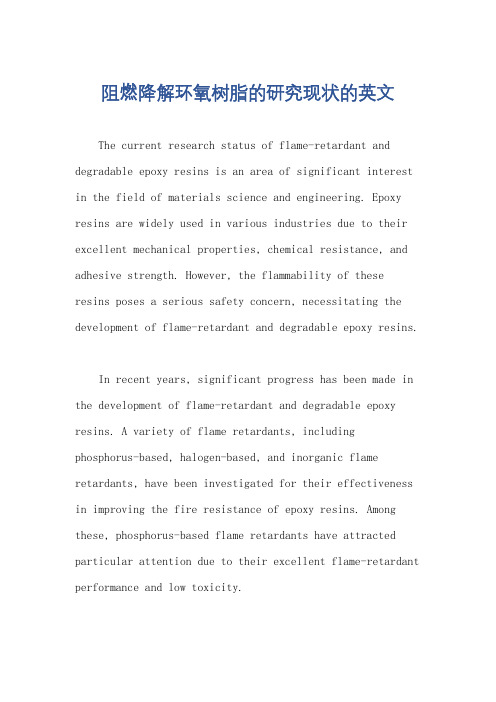
阻燃降解环氧树脂的研究现状的英文The current research status of flame-retardant and degradable epoxy resins is an area of significant interest in the field of materials science and engineering. Epoxy resins are widely used in various industries due to their excellent mechanical properties, chemical resistance, and adhesive strength. However, the flammability of these resins poses a serious safety concern, necessitating the development of flame-retardant and degradable epoxy resins.In recent years, significant progress has been made in the development of flame-retardant and degradable epoxy resins. A variety of flame retardants, including phosphorus-based, halogen-based, and inorganic flame retardants, have been investigated for their effectiveness in improving the fire resistance of epoxy resins. Among these, phosphorus-based flame retardants have attracted particular attention due to their excellent flame-retardant performance and low toxicity.In addition to flame retardants, biodegradable epoxy resins have also been developed to address the issue of waste disposal. These resins can be degraded under certain conditions, such as exposure to microorganisms or UV light, reducing their environmental impact. Biodegradable epoxy resins are typically based on renewable resources, such as vegetable oils or biobased monomers, which are derived from sustainable sources.The research on flame-retardant and degradable epoxy resins has focused on several key areas. One important aspect is the development of new flame retardants that can effectively improve the fire resistance of epoxy resins while maintaining their mechanical properties and processability. Another focus is on the design of novel degradation mechanisms that allow epoxy resins to degrade under specific conditions, such as exposure to microorganisms or UV light.Moreover, the integration of flame retardants and biodegradability into epoxy resins requires careful consideration of their compatibility and interactions. Thisinvolves studying the effects of flame retardants on the curing behavior, mechanical properties, and degradationrate of epoxy resins. Additionally, the stability and durability of flame-retardant and degradable epoxy resins under various environmental conditions need to be evaluated.In terms of applications, flame-retardant anddegradable epoxy resins have potential uses in a wide range of industries. They can be used in the electronics industry for the manufacture of flame-retardant circuit boards and insulation materials. In the construction industry, these resins can be employed in the development of fire-resistant coatings and flooring materials. Additionally, flame-retardant and degradable epoxy resins may find applications in the automotive, aerospace, and marine industries, where high-performance materials with excellent fire resistance and environmental compatibility are in demand.In conclusion, the research status of flame-retardant and degradable epoxy resins is promising. Significant progress has been made in the development of new flame retardants and degradation mechanisms, and the integrationof these features into epoxy resins is an active area of research. Future studies will focus on optimizing the performance of flame-retardant and degradable epoxy resins, exploring their potential applications, and addressing challenges related to compatibility, stability, and durability.。
反相微乳液模板法合成介孔聚苯乙烯

反相微乳液模板法合成介孔聚苯乙烯路霞唐静范玉冰胡军*刘洪来(华东理工大学化学系,结构可控先进功能材料及其制备教育部重点实验室,上海200237)摘要:提出并实施了反相微乳液为模板合成介孔聚合物的新方法.通过考察氯仿/CTAB/水三元系反相微乳液的稳定性,以及均相微乳液粒径变化规律,得到了三元反相微乳液体系的稳定区域.以(NH 4)2S 2O 8为引发剂,利用此反相微乳液为模板合成得到介孔聚苯乙烯材料.产物的XRD 图谱中有两个明显的衍射峰,对应的晶面间距离d 分别为2.6和1.3nm,1/d 值之比等于1∶2,为层状结构.N 2气吸附/脱附曲线表明大部分孔径为1.7nm,与动态光散射测得的微乳液液滴的尺寸相一致.关键词:介孔材料;聚苯乙烯;反相微乳液;模板;层状结构中图分类号:O648;O614Synthesis of Mesoporous Polystyrene Using Reversed MicroemulsionTemplate MethodLU XiaTANG JingFAN Yu -BingHU Jun *LIU Hong -Lai(Laboratory for Advanced Materials,Department of Chemistry,East China University of Science and Technology,Shanghai200237,P.R.China )Abstract :Using a reversed microemulsion as template,we developed a new and convenient synthesis method for mesoporous polymer materials.Based on a study of the stability and sizes of microemulsions at different mass ratios of CHCl 3/CTAB/H 2O,a stable reversed microemulsion region was discovered.The stable reversed microemulsion was used as a reaction system for the preparation of mesoporous polystyrene.The initiator (NH 4)2S 2O 8was dissolved in the reversed microemulsion ′s water core and polymerization of styrene was initiated at the oil -water interface of the microemulsion.With one intense and another weak diffraction peak,the synthesized polystyrene gave a typical XRD pattern for a lamellar mesostructure.The corresponding lamellar spacing of the (100)and (200)planes were 2.6and 1.3nm,respectively.The N 2adsorption/desorption isothermal suggested that the average pore size of the mesoporous polystyrene was mostly 1.7nm which coincided with the size of water drops in the reversed microemulsion.Key Words :Mesoporous material;Polystyrene;Reversed microemulsion;Template;Lamellar structure[Article]物理化学学报(Wuli Huaxue Xuebao )Acta Phys.-Chim.Sin .,2009,25(1):178-182Received:July 29,2008;Revised:October 10,2008;Published on Web:November 11,2008.*Corresponding author.Email:junhu@;Tel:+8621-64252921.国家自然科学基金(20776045,20736002),长江学者创新团队(IRT0721),111引智计划(B08021)和上海市自然科学基金(07ZR14029)资助项目鬁Editorial office of Acta Physico -Chimica Sinica1992年,Kreage 等[1]成功地合成出包括六方相MCM -41、立方相MCM -48和层状相MCM -50等具有规则孔道的介孔硅基分子筛,此后介孔材料已成为国内外学者研究的一个热点[2,3].介孔材料具有孔道规则有序、密度小、孔隙率高、比表面积大等优点[4],因其显著的表面效应在选择性吸附、形态选择性催化、分离等领域具有广阔的应用前景[5,6].迄今合成的介孔材料主要为无机材料,高分子有序介孔材料的合成尚处于探索阶段.由于高分子链的柔性和流动性,大部分合成体系为非水体系等特征,合成具有规则介观结构的高分子材料是一个巨大的挑战,无论是合成方法还是材料类型都十分有限.January178No.1路霞等:反相微乳液模板法合成介孔聚苯乙烯丰富多彩的高分子材料已成为这个世界不可缺少的部分,具有纳米或微米孔道的高分子材料兼具高分子材料本体的特性,又具有超高的表面效应,将在生物反应器、导电材料、传感器以及催化领域成为一类有着广阔应用前景的新型材料[7-10].在无机介孔材料合成中,软模板技术是重要手段之一,通常是以表面活性剂为模板剂,在水溶液中与无机前驱体通过界面反应,形成无机离子聚集体包覆于模板剂的有序组装体,通过焙烧或萃取方式除去模板剂后,留下无机骨架,从而获得无机介孔材料.通过改变表面活性剂的种类和用量,可以形成不同形状和尺寸的胶束模板,从而实现对孔结构的调控[11,12].而高分子多孔材料通常采用相分离法[13]和硬模板法[14]合成得到.所谓相分离法,即通过有机-有机组装中产生的微相分离,形成具有特定结构的高分子材料,通过浸蚀法或溶解嵌段共聚物(A-B)中的某一嵌段组分得到一定形貌的孔道结构.Chang等[15]研究了酚醛树脂、聚环氧乙烷(PEO)和聚ε-己酸内脂(PCL)三元共混物的相行为和组分之间氢键作用的关系.他们发现,虽然由这三个高分子组成的三对二元共混物均是完全互溶的,但由于三个组分之间氢键作用的差异,导致该三元共混物的相图中存在一个闭环区域,组成处于该区域的共混物是不混溶的,可以形成不同的介观分相结构.此项研究为后续的高分子介孔材料的研究提供了一定的理论指导.Hillmyer等[16]制成了一种规则的环氧树脂和两性嵌段共聚物的纳米混合物,但由于两性嵌段共聚物的除去存在困难,所以没有得到多孔的高分子材料.Gin等[17]合成出了一种有着规则孔道结构的介孔有机材料,但孔结构对热和溶剂的稳定性较差.近年来,合成无机介孔材料所采用的软模板技术也逐步成功应用于高分子介孔材料的合成,Zhao 等[18]采用三嵌段共聚物表面活性剂P123或F127为模板,以溶剂挥发诱导自组装方式合成了具有高度有序的六方相、立方相结构的介孔酚醛树脂,并通过高温焙烧,形成了介孔碳材料.Jang等[19]选用聚醚表面活性剂为模板剂,采用反胶束法合成了介孔聚丙烯腈,但该材料的孔道有序性较差.Nishiyama等[20]以间苯二酚/甲醛溶液(RF)和三乙氧基正已烷(EOA)为前驱体,F127为模板剂,在酸性条件下,400℃时F127分解,出现孔道结构,进一步在800℃下直接碳化合成出了规整的六方结构介孔碳材料COU-1.本文在软模板合成技术的基础上,提出了以有机溶剂/表面活性剂/水三元系形成的反相微乳液为反应介质,合成介孔高分子材料的新方法.采用氯仿(CH3Cl),十六烷基三甲基溴化铵(CTAB)和水三元反相微乳液为反应介质、(NH4)2S2O8为引发剂、苯乙烯为聚合物单体,二乙烯基苯为交联剂成功地合成了层状介孔聚苯乙烯,并对反相微乳液法合成介孔聚合物的机理进行了探讨.1实验部分1.1反相微乳液的研究将CTAB(分析纯,上海凌峰化学试剂有限公司)、CHCl3(分析纯,上海化学试剂有限公司)和去离子水(二次重蒸)配制成一系列不同质量比的溶液, m(CHCl3)/m(CTAB)/m(H2O)=7.5∶1.5∶m1(m1=0-4),超声振荡10min,混合均匀,然后分别在40℃恒温58h,考察水对反相微乳液稳定性的影响.同样配制质量比m(CHCl3)/m(CTAB)/m(H2O)=7.5∶m2∶0.3 (m2=0-3),考察CTAB对反相微乳液稳定性的影响.为更系统地考察三元反相微乳液的稳定性,固定CHCl3的量,在油包水反相微乳液范围内随机加入水和表面活性剂,配制成系列不同质量比的溶液.1.2粒径测定采用英国马尔文公司Nano-ZS型动态光散射仪在40℃下测定溶液中反相微乳液的粒径,激光源He-Ne,4.0mV,633nm,动态光散射-非侵入式背散射技术.1.3介孔聚苯乙烯的合成将过硫酸铵(NH4)2S2O8(分析纯,上海凌峰化学试剂有限公司)溶于水中,并与适量比例的CTAB和CHCl3混溶,超声混合10min,然后在40℃下恒温搅拌2h.将苯乙烯和二乙烯基苯加入反应体系中,继续搅拌2min后停止搅拌,静置.反应进行若干时间后,得到白色聚苯乙烯样品.将上述聚苯乙烯样品1g,加入乙醇100mL,盐酸18mL,70℃回流8h,提取模板剂.然后抽滤,常温真空干燥24h. 1.4介孔苯乙烯的表征XRD测试在日本理学D/Max2550VB/PC型X射线粉末衍射仪上进行,管电压40kV,管电流200mA,铜靶,小角扫描区间为1°-10°;吸附/脱附等温线在Micrometrics ASAP2020型物理吸附仪上测量,吸附质为N2,温度为-196℃,样品的孔径分布由BJH法处理得到.179Acta Phys.-Chim.Sin.,2009Vol.252结果与讨论2.1CHCl 3/CTAB/H 2O 反相微乳液CHCl 3/CTAB/H 2O 三元系统在适当的配比下可形成均一稳定的微乳液.本文采用的高分子单体能溶于CHCl 3,为了合成有序的具有介观结构的聚合物材料,必须选择CHCl 3为连续相的油包水型反向微乳液系统,即CTAB 反胶束中包裹了微量的水.40℃下,氯仿、水和表面活性剂的三元局部相图如图1所示.图中坐标单位均为质量分数,曲线所围内部为均相区.l 1线为保持氯仿和水的质量比不变,考察表面活性剂量的变化对系统稳定性的影响.当CTAB 的含量比较少时,形成的反胶束还不足以容纳所有的水,因此游离在胶束外的水和氯仿形成互不相溶的两相.随着CTAB 量增加,形成的反胶束量增多,当足以包裹全部水时,反相微乳液系统为均相.保持氯仿和CTAB 质量比不变,考察水量变化的影响,如l 2线,随水含量的上升,系统由均相变为非均相.合成聚合物介孔材料所需的反相微乳液反应体系处在均相区域内.反相微乳液的物理性质主要取决其含水量W 0,W 0=C (H 2O)/C (surfactant),其中C 为摩尔浓度.W 0决定了反相微乳液滴的大小和每个液滴中所含表面活性剂的数目.图2是反相微乳液粒径随含水量W 0的变化情况.当W 0较小时,少量的水被包裹在反相微乳液的内核中,微乳液粒径基本不变,为1.7nm 左右.W 0大于7.0,反相微乳液液滴随水含量的增加,粒径有显著的增加.可以预计,随着W 0继续增加,反相微乳液系统将失去稳定性.2.2介孔聚苯乙烯的表征选取上述W 0<7.0的稳定微乳液体系,通过微乳液诱导、自由基聚合得到了白色粉末状聚苯乙烯.其XRD 图谱如图3所示,在衍射角2θ=3.3°时出现明显的衍射峰1,表明其具有一定的介观结构.同时在2θ=6.7°处还有一弱衍射峰2,根据布拉格公式2d sin θ=n λ(n =1)(式中d 为晶面间距离,θ为衍射角,n 为衍射级数,λ为X 射线波长),衍射峰对应的晶面间距离d 分别为2.6和1.3nm,相应衍射晶面分别为(100)、(200),1/d 值的比为1∶2,为典型的层状相[21].由此可以判定合成产物聚苯乙烯具有层状介观结构.此外,衍射峰1前的微小峰为CTAB 特征峰,表明还有微量CTAB 没有除尽.层状结构的聚苯乙烯对N 2气的吸附/脱附曲线如图4(a)所示,为典型的II 型吸附/脱附线,吸附量在较低相对压力(p /p 0)时迅速增加,在中间相对压力时,吸附量继续升高,但变化不明显,几乎平行于相对压力坐标轴,表明孔径较小,在相对压力趋图2反相微乳液粒径(d )随含水量W 0的变化Fig.2Size (d )changes of reversed microemulsionwith W 0W 0=C (H 2O)/C (surfactant)图3聚苯乙烯的XRD 图Fig.3XRD pattern of PS mesoporous material图140℃时,CHCl 3/CTAB/H 2O 系统三元相图Fig.1Ternary phase diagram of the CHCl 3/CTAB/H 2O system at 40℃■represents the homogeneous phase,and ▲represents theheterogeneous phase.w H 2Ow CT A B→→→180No.1路霞等:反相微乳液模板法合成介孔聚苯乙烯近于1时,吸附量增加,没有出现吸附极限,可能是材料外表面吸附所致.氮气的总体吸附量较小,表明材料的比表面积较小.这可能有两种原因引起,其一,由于聚合物链具有一定的柔性,模板剂提取后致使聚合物层状结构坍塌;其二,由于聚合物链具有一定的流动性,模板剂提取过程中溶剂的热回流致使部分孔道口被游离的聚合物链堵塞.部分结构的坍塌和孔道堵塞致使N2气吸附分析中,气体无法进入内孔道,氮气的总体吸附量较小.通过BJH 模型对脱附曲线进行计算,得到的孔径分布如图4 (b),平均孔径为7nm,其中大部分孔径为1.7nm,与上述微乳液液滴的尺寸相匹配.进一步证明聚苯乙烯的层状结构是由反向微乳液模板诱导形成的.2.3反相微乳液模板法合成介孔聚苯乙烯的机理实验结果表明利用反相微乳液合成介孔聚合物的设计方法具有可行性,为使反相微乳液法成为简单而通用的合成介孔聚合物的新方法,探讨其合成机理是极为必要的.图5给出了采用CHCl3/ CTAB/H2O反相微乳液系统合成介孔聚苯乙烯的机理.包含四个步骤:微乳液形成→界面引发→聚合→除模板.如图5(a)所示,在一定配比下CHCl3/CTAB/H2O 三元系统形成反相微乳液滴,CTAB头基聚集在水相,尾链伸展于CHCl3油相中.水溶性引发剂过硫酸图4聚苯乙烯的(a)N2气吸附/脱附曲线和(b)采用BJH模型处理脱附曲线得到的孔径分布曲线Fig.4(a)Adsorption and desorption isotherm of N2on PS mesopourous material,(b)the pore size distributioncurve by the BJH analysis of desorption branch isothermH2O Oil图5由反向微乳液合成介孔聚苯乙烯的机理图Fig.5Schematic procedure of synthesis of mesoporous PS by the reversed microemulsion template181Acta Phys.-Chim.Sin.,2009Vol.25铵(NH4)2S2O8被CTAB包裹在水滴内核,相互碰撞发生反应形成自由基.聚合物单体苯乙烯和二乙烯基苯溶解在CHCl3油相中,在反相微乳液CTAB-油相界面的苯乙烯和二乙烯基苯单体捕获到(NH4)2S2O8分解产生的自由基,从而引发自由基聚合反应,如图5(b)所示.自由基既可能沿着微乳液表面传递,在界面生成聚合物;也可能逐步向油相传递,在油相生成连续的聚合物;其中二乙烯基苯起交联剂作用,使油相中的聚合物形成网状结构,从而保留并固定微乳液模板剂的结构,如图5(c)所示.聚合反应结束后,通过乙醇破乳,并除去表面活性剂模板,即得到聚合物母体中含有规则孔道的介孔聚合物材料,如图5(d).介孔聚合物材料的介孔结构决定于模板剂的结构,但单体在聚合过程中有可能通过挤压、聚集等相互作用方式,改变软模板的结构形貌,最终获得的聚合物介孔结构与初始的模板结构不一定完全相同.聚合过程对介孔结构的影响有待进一步研究.3结论在合适的比例下CHCl3/CTAB/H2O三元系可以形成均一的反相微乳液.在含水量W0<7.0时,此反相微乳液稳定,液滴粒径为1.7nm左右基本不变.继续增加W0,反相微乳液系统失去稳定性.以稳定的均一反相微乳液为模板,水溶性(NH4)2S2O8为引发剂,通过界面引发、体相聚合形成了聚苯乙烯材料,产物XRD图有两个明显的衍射峰,其1/d值比为1∶2,表明具有层状结构,其(100)面层间距离为2.6nm.N2气吸附/脱附曲线表明大部分孔道直径为1.7nm.以反相微乳液为模板合成介孔聚合物的机理包含四个步骤:微乳液形成→界面引发→聚合→除模板,该机理为简便合成介孔聚合物提供了新思路.References1Kreage,C.T.;Leonowicz,M.E.;Roth,W.J.;Vartuli,J.C.;Beck, J.S.Nature,1992,359:7102Impens,N.R.E.N.;van der Voort,P.;Vansant,E.F.Micropor.Mesopor.Mater.,1999,28:2173Zhang,Z.R.;Suo,J.S.;Zhang,X.M.;Li,S.B.Progress in Chemistry,1999,11(1):11[张兆荣,索继栓,张小明,李树本.化学进展,1999,11(1):11]4Li,Y.;Kim,S.J.J.Phys.Chem.B,2005,109:123095Ryoo,R.;Joo,S.H.;Yun,S.J.Phys.Chem.B,1999,103:77436Attard,G.S.;Bartlett,P.N.;Coleman,N.R.B.;Elliott,J.M.;Owen,J.R.;Wang,J.H.Science,1997,278:23737Green,P.F.;Limary,R.Adv.Colloid Interface,2001,94:538Peters,E.;Svec,C.F.;J.Frechet,M.J.Adv.Mater.,1999,11: 11699Hedrick,J.L.;Miller,R.D.;Hawker,C.J.;Carter,K.R.;Volksen, W.;Yoon,D.Y.;Trollsas,M.Adv.Mater.,1998,10:104910de Boer,B.;Stalmach,U.;Nijland,H.;Hadziioannou,G.Adv.Mater.,2000,12:158111Fajula,F.;Galarneau,A.;Renzo,F.D.Micropor.Mesopor.Mater., 2005,82:22712Hu,J.;Zhou,L.H.;Li,H.N.;Li,W.X.;Liu,H.L.;Hu,Y.Acta Phys.-Chim.Sin.,2005,21(11):1217[胡军,周丽绘,李鸿宁,李万鑫,刘洪来,胡英.物理化学学报,2005,21(11):1217]13Jenekhe,S.A.;Chen,X.L.Science,1999,283:37214Johnson,S.A.;Ollivier,P.J.;Mallouk,T.E.Science,1999,283: 96315Kuo,S.W.;Lin,C.L.;Chang,F.C.Macromolecules,2002,35: 27816Lipic,P.M.;Bates,F.S.;Hillmyer,M.A.J.Am.Chem.Soc., 1998,120:896317Miller,S.A.;Kim,E.;Gray,D.H.;Gin,D.L.Angew.Chem.Int.Ed.,1999,38(20):302118Meng,Y.;Gu,D.;Zhang,F.Q.;Shi,Y.F.;Yang,H.F.;Li,Z.;Yu,C.Z.;Tu,B.;Zhao,D.Y.Angew.Chem.Int.Ed.,2005,44:7053 19Jang,J.;Bae,mun.,2005,(9):120020Tanaka,S.;Nishiyama,N.;Egashira,Y.;Ueyama,K.Chem.Commun.,2005,(16):212521Ma,L.D.Modern X-ray powder diffraction.Beijing:Chemical Industrial Press,2004:16-90[马礼敦.近代X射线多晶体衍射.北京:化学工业出版社,2004:16-90]182。
高分子专业术语中英文对照表
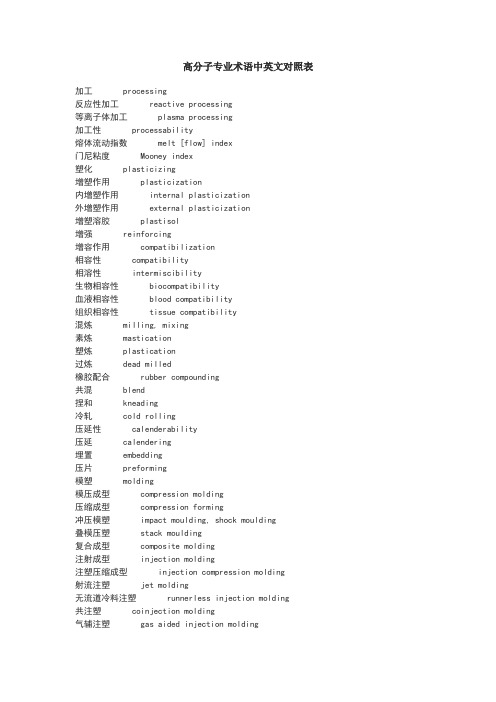
高分子专业术语中英文对照表加工 processing反应性加工 reactive processing等离子体加工 plasma processing加工性 processability熔体流动指数 melt [flow] index门尼粘度 Mooney index塑化 plasticizing增塑作用 plasticization内增塑作用 internal plasticization外增塑作用 external plasticization增塑溶胶 plastisol增强 reinforcing增容作用 compatibilization相容性 compatibility相溶性 intermiscibility生物相容性 biocompatibility血液相容性 blood compatibility组织相容性 tissue compatibility混炼 milling, mixing素炼 mastication塑炼 plastication过炼 dead milled橡胶配合 rubber compounding共混 blend捏和 kneading冷轧 cold rolling压延性 calenderability压延 calendering埋置 embedding压片 preforming模塑 molding模压成型 compression molding压缩成型 compression forming冲压模塑 impact moulding, shock moulding叠模压塑 stack moulding复合成型 composite molding注射成型 injection molding注塑压缩成型 injection compression molding射流注塑 jet molding无流道冷料注塑 runnerless injection molding共注塑 coinjection molding气辅注塑 gas aided injection molding注塑焊接 injection welding传递成型 transfer molding树脂传递成型 resin transfer molding铸塑 cast熔铸 fusion casting铸塑成型 cast molding单体浇铸 monomer casting挤出 extrusion共挤出 coextrusion多层挤塑 multi-layer extrusion共挤吹塑 coextrusion blow molding同轴挤塑 coaxial extrusion吹胀挤塑 blown extrusion挤出吹塑 extrusion blow molding挤拉吹塑成型 extrusion draw blow molding反应性挤塑 reactive extrusion固相挤出 solid-phase extrusion发泡 expanding foam后发泡 post expansion物理发泡 physical foam化学发泡 chemical foam吹塑 blow molding多层吹塑 multi-layer blow molding拉伸吹塑成型 stretch blow molding滚塑 rotational moulding反应注射成型 reaction injection molding, RIM 真空成型 vacuum forming无压成型 zero ressure molding真空烧结 vacuum sintering真空袋成型 vacuum bag molding热成型 thermal forming拉伸热成型 stretch thermoforming袋模塑 bag molding糊塑 paste molding镶铸 imbedding冲压成型 impact molding触压成型 impression molding层压材料 laminate泡沫塑料成型 foam molding包模成型 drape molding充气吹胀 inflation橡胶胶乳 rubber latex胶乳 latex高分子胶体 polymer colloid生橡胶 raw rubber,crude rubber硬质胶 ebonite再生胶 reclaimed rubber充油橡胶 oil-extended rubber母胶 masterbatch交联 crosslinking固化 cure光固化 photo-cure硫化 vulcanization后硫化 post cure,post vulcanization自硫[化] bin cure自交联 self crosslinking , self curing过硫 over cure返硫 reversion欠硫 under cure动态硫化 dynamic vulcanization不均匀硫化 heterogeneous vulcanization开始[硫化]效应 set-up effect自动硫化 self-curing, self-vulcanizing焦烧 scorching无压硫化 non-pressure cure模压硫化 moulding curing常温硫化 auto-vulcanization热硫化 heat curing蒸汽硫化 steam curing微波硫化 micro wave curing辐射硫化 radiation vulcanization辐射交联 radiation crosslinking连续硫化 continuous vulcanization无模硫化 open vulcanization成纤 fiber forming可纺性 spinnability纺丝 spinning干纺 dry spinning湿纺 wet spinning干湿法纺丝 dry wet spinning干喷湿法纺丝 dry jet wet spinning溶液纺丝 solution spinning乳液纺丝 emulsion spinning乳液闪蒸纺丝法 emulsion flash spinning process 喷射纺丝 jet spinning喷纺成形 spray spinning液晶纺丝 liquid crystal spinning熔纺 melt spinning共混纺丝 blended spinning凝胶纺[丝] gel spinning反应纺丝 reaction spinning静电纺丝 electrostatic spinning高压纺丝 high-pressure spinning复合纺丝 conjugate spinning无纺布 non-woven fabrics单丝 monofilament, monofil复丝 multifilament全取向丝 fully oriented yarn中空纤维 hollow fiber皮芯纤维 sheath core fiber共纺 cospinning冷拉伸 cold drawing, cold stretching 单轴拉伸 uniaxial drawing,uniaxial elongation双轴拉伸 biaxial drawing多轴拉伸 multiaxial drawing皮心效应 skin and core effect皮层效应 skin effect防缩 non-shrink熟成 ripening垂挂 sag定型 sizing起球现象 pilling effect捻度 twist旦 denier特 tex纱 yarn股 strand粘合 adhesion反应粘合 reaction bonding压敏粘合 pressure sensitive adhesion 底漆 primer浸渍 impregnation浸渍树脂 solvent impregnated resin基体 matrix聚合物表面活性剂 polymeric surfactant 高分子絮凝剂 polymeric flocculant预发颗粒 pre-expanded bead高分子膜 polymeric membraneH-膜 H-filmLB膜 Langmuir Blodgett film (LB film) 半透膜 semipermeable membrane反渗透膜 Reverse osmosis membrance多孔膜 porous membrane各向异性膜 anisotropic membrane正离子交换膜 cation exchange membrane负离子交换膜 anionic exchange membrane 吸附树脂 polymeric adsorbent添加剂 additive固化剂 curing agent潜固化剂 latent curing agent硫化剂 vulcanizing agent给硫剂 sulfur donor agent, sulfur donor 硫化促进剂 vulcanization accelerator硫化活化剂 vulcanization activator活化促进剂 activating accelerator活化剂 activator防焦剂 scorch retarder抗硫化返原剂 anti-reversion agent塑解剂 peptizer偶联剂 coupling agent硅烷偶联剂 silane coupling agent钛酸酯偶联剂 titanate coupling agent铝酸酯偶联剂 aluminate coupling agent 增强剂 reinforcing agent增硬剂 hardening agent惰性填料 inert filler增塑剂 plasticizer辅增塑剂 coplasticizer增粘剂 tackifier增容剂 compatibilizer增塑增容剂 plasticizer extender分散剂 dispersant agent结构控制剂 constitution controller色料 colorant荧光增白剂 optical bleaching agent抗降解剂 antidegradant防老剂 anti-aging agent防臭氧剂 antiozonant抗龟裂剂 anticracking agent抗疲劳剂 anti-fatigue agent抗微生物剂 biocide防蚀剂 anti-corrosion agent光致抗蚀剂 photoresist防霉剂 antiseptic防腐剂 rot resistor防潮剂 moisture proof agent除臭剂 re-odorant抗氧剂 antioxidant热稳定剂 heat stabilizer抗静电添加剂 antistatic additive抗静电剂 antistatic agent紫外线稳定剂 ultraviolet stabilizer紫外光吸收剂 ultraviolet absorber光稳定剂 light stabilizer, photostabilizer 光屏蔽剂 light screener发泡剂 foaming agent物理发泡剂 physical foaming agent化学发泡剂 chemical foaming agent脱模剂 releasing agent内脱模剂 internal releasing agent外脱模剂 external releasing agent阻燃剂 flame retardant防火剂 fire retardant烧蚀剂 ablator润滑剂 lubricant湿润剂 wetting agent隔离剂 separant增韧剂 toughening agent抗冲改性剂 impact modifier消泡剂 antifoaming agent减阻剂 drag reducer破乳剂 demulsifier粘度改进剂 viscosity modifier增稠剂 thickening agent, thickener阻黏剂 abhesive洗脱剂 eluant附聚剂 agglomerating agent后处理剂 after-treating agent催干剂 drier防结皮剂 anti-skinning agent纺织品整理剂 textile finishing agent-----------------------高物高化类:结构单元 constitutional unit重复结构单元 constitutional repeating unit 构型单元 configurational unit立构重复单元 stereorepeating unit立构规整度 tacticity等规度, 全同立构[规整]度 isotacticity间同度,间同立构[规整]度 syndiotacticity无规度,无规立构度 atacticity嵌段 block规整嵌段 regular block非规整嵌段 irregular block立构嵌段 stereoblock有规立构嵌段 isotactic block无规立构嵌段 atactic block单体单元 monomeric unit二单元组 diad三单元组 triad四单元组 tetrad五单元组 pentad无规线团 random coil自由连接链 freely-jointed chain自由旋转链 freely-rotating chain蠕虫状链 worm-like chain柔性链 flexible chain链柔性 chain flexibility刚性链 rigid chain棒状链 rodlike chain链刚性 chain rigidity聚集 aggregation聚集体 aggregate凝聚、聚集 coalescence链缠结 chain entanglement凝聚缠结 cohesional entanglement物理缠结 physical entanglement拓扑缠结 topological entanglement凝聚相 condensed phase凝聚态 condensed state凝聚过程 condensing process临界聚集浓度 critical aggregation concentration 线团-球粒转换 coil-globule transition受限链 confined chain受限态 confined state物理交联 physical crosslinking统计线团 statistical coil等效链 equivalent chain统计链段 statistical segment链段 chain segment链构象 chain conformation无规线团模型 random coil model无规行走模型 random walk model自避随机行走模型 self avoiding walk model卷曲构象 coiled conformation高斯链 Gaussian chain无扰尺寸 unperturbed dimension扰动尺寸 perturbed dimension热力学等效球 thermodynamically equivalent sphere近程分子内相互作用 short-range intramolecular interaction远程分子内相互作用 long-range intramolecular interaction链间相互作用 interchain interaction链间距 interchain spacing长程有序 long range order近程有序 short range order回转半径 radius of gyration末端间矢量 end-to-end vector链末端 chain end末端距 end-to-end distance无扰末端距 unperturbed end-to-end distance均方根末端距 root-mean-square end-to-end distance伸直长度 contour length相关长度 persistence length主链;链骨架 chain backbone支链 branch chain链支化 chain branching短支链 short-chain branch长支链 long-chain branch支化系数 branching index支化密度 branching density支化度 degree of branching交联度 degree of crosslinking网络 network网络密度 network density溶胀 swelling平衡溶胀 equilibrium swelling分子组装,分子组合 molecular assembly自组装 self assembly微凝胶 microgel凝胶点 gel point可逆[性]凝胶 reversible gel溶胶-凝胶转化 sol-gel transformation临界胶束浓度 critical micelle concentration,CMC组成非均一性 constitutional heterogenity, compositional heterogenity 摩尔质量平均 molar mass average数均分子量 number-average molecular weight, number-average molar mass重均分子量 weight-average molecular weight, weight-average molar mass Z均分子量 Z(Zaverage)-average molecular weight, Z-molar mass黏均分子量 viscosity-average molecular weight,viscosity-average molar mass表观摩尔质量 apparent molar mass表观分子量 apparent molecular weight聚合度 degree of polymerization动力学链长 kinetic chain length单分散性 monodispersity临界分子量 critical molecular weight分子量分布 molecular weight distribution,MWD多分散性指数 polydispersity index,PID平均聚合度 average degree of polymerization质量分布函数 mass distribution function数量分布函数 number distribution function重量分布函数 weight distribution function舒尔茨-齐姆分布 Schulz-Zimm distribution最概然分布 most probable distribution对数正态分布 logarithmic normal distribution聚合物溶液 polymer solution聚合物-溶剂相互作用 polymer-solvent interaction溶剂热力学性质 thermodynamic quality of solvent均方末端距 mean square end to end distance均方旋转半径 mean square radius of gyrationθ温度 theta temperatureθ态 theta stateθ溶剂 theta solvent良溶剂 good solvent不良溶剂 poor solvent位力系数 Virial coefficient排除体积 excluded volume溶胀因子 expansion factor溶胀度 degree of swelling弗洛里-哈金斯理论 Flory-Huggins theory哈金斯公式 Huggins equation哈金斯系数 Huggins coefficientχ(相互作用)参数χ-parameter溶度参数 solubility parameter摩擦系数 frictional coefficient流体力学等效球 hydrodynamically equivalent sphere流体力学体积 hydrodynamic volume珠-棒模型 bead-rod model球-簧链模型 ball-spring [chain] model流动双折射 flow birefringence, streaming birefringence动态光散射 dynamic light scattering小角激光光散射 low angle laser light scattering沉降平衡 sedimentation equilibrium沉降系数 sedimentation coefficient沉降速度法 sedimentation velocity method沉降平衡法 sedimentation equilibrium method相对黏度 relative viscosity相对黏度增量 relative viscosity increment黏度比 viscosity ratio黏数 viscosity number[乌氏]稀释黏度计 [Ubbelohde] dilution viscometer毛细管黏度计 capillary viscometer落球黏度计 ball viscometer落球黏度 ball viscosity本体黏度 bulk viscosity比浓黏度 reduced viscosity比浓对数黏度 inherent viscosity, logarithmic viscosity number 特性黏数 intrinsic viscosity, limiting viscosity number黏度函数 viscosity function零切变速率黏度 zero shear viscosity端基分析 analysis of end group蒸气压渗透法 vapor pressure osmometry, VPO辐射的相干弹性散射 coherent elastic scattering of radiation 折光指数增量 refractive index increment瑞利比 Rayleigh ratio超瑞利比 excess Rayleigh ratio粒子散射函数 particle scattering function粒子散射因子 particle scattering factor齐姆图 Zimm plot散射的非对称性 dissymmetry of scattering解偏振作用 depolarization分级 fractionation沉淀分级 precipitation fractionation萃取分级 extraction fractionation色谱分级 chromatographic fractionation柱分级 column fractionation洗脱分级,淋洗分级 elution fractionation热分级 thermal fractionation凝胶色谱法 gel chromatography摩尔质量排除极限 molar mass exclusion limit溶剂梯度洗脱色谱法 solvent gradient [elution] chromatography 分子量排除极限 molecular weight exclusion limit洗脱体积 elution volume普适标定 universal calibration加宽函数 spreading function链轴 chain axis等同周期 identity period链重复距离 chain repeating distance晶体折叠周期 crystalline fold period构象重复单元 conformational repeating unit几何等效 geometrical equivalence螺旋链 helix chain构型无序 configurational disorder链取向无序 chain orientational disorder构象无序 conformational disorder锯齿链 zigzag chain双[股]螺旋 double stranded helix[分子]链大尺度取向 global chain orientation结晶聚合物 crystalline polymer半结晶聚合物 semi-crystalline polymer高分子晶体 polymer crystal高分子微晶 polymer crystallite结晶度 degree of crystallinity, crystallinity 高分子[异质]同晶现象 macromolecular isomorphism 聚合物形态学 morphology of polymer片晶 lamella, lamellar crystal轴晶 axialite树枝[状]晶体 dendrite纤维晶 fibrous crystal串晶结构 shish-kebab structure球晶 spherulite折叠链 folded chain链折叠 chain folding折叠表面 fold surface折叠面 fold plane折叠微区 fold domain相邻再入模型 adjacent re-entry model接线板模型 switchboard model缨状微束模型 fringed-micelle model折叠链晶体 folded-chain crystal平行链晶体 parallel-chain crystal伸展链晶体 extended-chain crystal球状链晶体 globular-chain crystal长周期 long period近程结构 short-range structure远程结构 long-range structure成核作用 nucleation分子成核作用 molecular nucleation阿夫拉米方程 Avrami equation主结晶 primary crystallization后期结晶 secondary crystallization外延结晶,附生结晶 epitaxial crystallization外延晶体生长,附生晶体生长 epitaxial growth织构 texture液晶态 liquid crystal state溶致性液晶 lyotopic liquid crystal热致性液晶 thermotropic liquid crystal热致性介晶 thermotropic mesomorphism近晶相液晶 smectic liquid crystal近晶中介相 smectic mesophase近晶相 smectic phase条带织构 banded texture环带球晶 ringed spherulite向列相 nematic phase盘状相 discotic phase解取向 disorientation分聚 segregation非晶相 amorphous phase非晶区 amorphous region非晶态 amorphous state非晶取向 amorphous orientation链段运动 segmental motion亚稳态 metastable state相分离 phase separation亚稳相分离 spinodal decompositionbimodal decomposition微相 microphase界面相 boundary phase相容性 compatibility混容性 miscibility不相容性 incompatibility不混容性 immiscibility增容作用 compatiibilization最低临界共溶(溶解)温度 lower critical solution temperature, LCST 最高临界共溶(溶解)温度 upper critical solution temperature , UCST 浓度猝灭 concentration quenching激基缔合物荧光 excimer fluorescence激基复合物荧光 exciplex fluorescence激光共聚焦荧光显微镜 laser confocal fluorescence microscopy单轴取向 uniaxial orientation双轴取向 biaxial orientation, biorientation取向度 degree of orientation橡胶态 rubber state玻璃态 glassy state高弹态 elastomeric state黏流态 viscous flow state伸长 elongation高弹形变 high elastic deformation回缩性,弹性复原 nerviness拉伸比 draw ratio, extension ratio泊松比 Poisson's ratio杨氏模量 Young's modulus本体模量 bulk modulus剪切模量 shear modulus法向应力 normal stress剪切应力 shear stress剪切应变 shear strain屈服 yielding颈缩现象 necking屈服应力 yield stress屈服应变 yield strain脆性断裂 brittle fracture脆性开裂 brittle cracking脆-韧转变 brittle ductile transition脆化温度 brittleness(brittle) temperature延性破裂 ductile fracture冲击强度 impact strength拉伸强度 tensile strength极限拉伸强度 ultimate tensile strength抗撕强度 tearing strength弯曲强度 flexural strength, bending strength 弯曲模量 bending modulus弯曲应变 bending strain弯曲应力 bending stress收缩开裂 shrinkage crack剪切强度 shear strength剥离强度 peeling strength疲劳强度 fatigue strength, fatigue resistance 挠曲 deflection压缩强度 compressive strength压缩永久变形 compression set压缩变形 compressive deformation压痕硬度 indentation hardness洛氏硬度 Rockwell hardness布氏硬度 Brinell hardness抗刮性 scrath resistance断裂力学 fracture mechanics力学破坏 mechanical failure应力强度因子 stress intensity factor断裂伸长 elongation at break屈服强度 yield strength断裂韧性 fracture toughness弹性形变 elastic deformation弹性滞后 elastic hysteresis弹性 elasticity弹性模量 modulus of elasticity弹性回复 elastic recovery不可回复形变 irrecoverable deformation 裂缝 crack银纹 craze形变;变形 deformation永久变形 deformation set剩余变形 residual deformation剩余伸长 residual stretch回弹,回弹性 resilience延迟形变 retarded deformation延迟弹性 retarded elasticity可逆形变 reversible deformation应力开裂 stress cracking应力-应变曲线 stress strain curve拉伸应变 stretching strain拉伸应力弛豫 tensile stress relaxation 热历史 thermal history热收缩 thermoshrinking扭辫分析 torsional braid analysis,TBA 应力致白 stress whitening应变能 strain energy应变张量 strain tensor剩余应力 residual stress应变硬化 strain hardening应变软化 strain softening电流变液 electrorheological fluid假塑性 pseudoplastic拉胀性 auxiticity牛顿流体 Newtonian fluid非牛顿流体 non-Newtonian fluid宾汉姆流体 Bingham fluid冷流 cold flow牛顿剪切黏度 Newtonian shear viscosity 剪切黏度 shear viscosity表观剪切黏度 apparent shear viscosity剪切变稀 shear thinning触变性 thixotropy塑性形变 plastic deformation塑性流动 plastic flow体积弛豫 volume relaxation拉伸黏度 extensional viscosity黏弹性 viscoelasticity线性黏弹性 linear viscoelasticity非线性黏弹性 non-linear viscoelasticity蠕变 creep弛豫[作用] relaxation弛豫模量 relaxation modulus蠕变柔量 creep compliance热畸变温度 heat distortion temperature弛豫谱 relaxation spectrum推迟[时间]谱 retardation [time] spectrum弛豫时间 relaxation time推迟时间 retardation time动态力学行为 dynamic mechanical behavior动态黏弹性 dynamic viscoelasticity热-机械曲线 thermo-mechanical curve动态转变 dynamic transition储能模量 storage modulus损耗模量 loss modulus复数模量 complex modulus复数柔量 complex compliance动态黏度 dynamic viscosity复数黏度 complex viscosity复数介电常数 complex dielectric permittivity介电损耗因子 dielectric dissipation factor介电损耗常数 dielectric loss constant介电弛豫时间 dielectric relaxation time玻璃化转变 glass transition玻璃化转变温度 glass-transition temperature次级弛豫 secondary relaxation次级转变 secondary transition次级弛豫温度 secondary relaxation temperature开尔文模型 Kelvin model麦克斯韦模型 Maxwell model时-温叠加原理 time-temperature superposition principle 玻耳兹曼叠加原理 Boltzmann superposition principle平移因子 shift factorWLF公式 WLF[Williams-Lendel-Ferry] equation软化温度 softening temperature平衡熔点 equilibrium melting point物理老化 physical ageing光老化 photoageing热老化 thermal ageing热氧老化 thermo-oxidative ageing人工老化 artificial ageing加速老化 accelerated ageing计算机模拟 computer simulation分子动力学模拟 molecular dynamics simulation蒙特卡洛模拟 Monte Carlo simulation---------------------------------聚合反应类:单体 monomer官能度 functionality平均官能度 average functionality双官能[基]单体 bifunctional monomer三官能[基]单体 trifunctional monomer乙烯基单体 vinyl monomer1,1-亚乙烯基单体,偏[二]取代乙烯单体 vinylidene monomer1,2-亚乙烯基单体,1,2-二取代乙烯单体 vinylene monomer双烯单体,二烯单体 diene monomer极性单体 polar monomer非极性单体 non polar monomer共轭单体 conjugated monomer非共轭单体 non conjugated monomer活化单体 activated monomer官能单体 functional monomer大分子单体 macromer, macromonomer环状单体 cyclic monomer共聚单体 comonomer聚合[反应] polymerization均聚反应 homopolymerization低聚反应,齐聚反应 (曾用名) oligomerization调聚反应 telomerization自发聚合 spontaneous polymerization预聚合 prepolymerization后聚合 post polymerization再聚合 repolymerization铸塑聚合, 浇铸聚合 cast polymerization链[式]聚合 chain polymerization烯类聚合,乙烯基聚合 vinyl polymerization双烯[类]聚合 diene polymerization加[成]聚[合] addition polymerization自由基聚合,游离基聚合 (曾用名) free radical polymerization,radical polymerization控制自由基聚合,可控自由基聚合 controlled radical polymerization,CRP活性自由基聚合 living radical polymerization原子转移自由基聚合 atom transfer radical polymerization,ATRP反向原子转移自由基聚合 reverse atom transfer radical polymerization, RATRP 可逆加成断裂链转移 reversible addition fragmentation chaintransfer,RAFT氮氧[自由基]调控聚合 nitroxide mediated polymerization稳定自由基聚合 stable free radical polymerization,FRP自由基异构化聚合 free radical isomerization polymerization自由基开环聚合 radical ring opening polymerization氧化还原聚合 redox polymerization无活性端聚合,死端聚合 (曾用名) dead end polymerization光[致]聚合 photo polymerization光引发聚合 light initiated polymerization光敏聚合 photosensitized polymerization四中心聚合 four center polymerization电荷转移聚合 charge transfer polymerization辐射引发聚合 radiation initiated polymerization热聚合 thermal polymerization电解聚合 electrolytic polymerization等离子体聚合 plasma polymerization易位聚合 metathesis polymerization开环易位聚合 ring opening metathesis polymerization,ROMP精密聚合 precision polymerization环化聚合 cyclopolymerization拓扑化学聚合 topochemical polymerization平衡聚合 equilibrium polymerization离子[型]聚合 ionic polymerization辐射离子聚合 radiation ion polymerization离子对聚合 ion pair polymerization正离子聚合,阳离子聚合 cationic polymerization碳正离子聚合 carbenium ion polymerization,carbocationic polymerization假正离子聚合 pseudo cationic polymerization假正离子活[性]聚合 pseudo cationic living polymerization活性正离子聚合 living cationic polymerization负离子聚合,阴离子聚合 anionic polymerization碳负离子聚合 carbanionic polymerization活性负离子聚合 living anionic polymerization负离子环化聚合 anionic cyclopolymerization负离子电化学聚合 anionic electrochemical polymerization负离子异构化聚合 anionic isomerization polymerization烯丙基聚合 allylic polymerization活[性]聚合 living polymerization两性离子聚合 zwitterion polymerization齐格勒-纳塔聚合 Ziegler Natta polymerization配位聚合 coordination polymerization配位离子聚合 coordinated ionic polymerization配位负离子聚合 coordinated anionic polymerization配位正离子聚合 coordinated cationic polymerization插入聚合 insertion polymerization定向聚合,立构规整聚合 stereoregular polymerization, stereospecific polymerization 有规立构聚合 tactic polymerization全同立构聚合 isospecific polymerization不对称诱导聚合 asymmetric induction polymerization不对称选择性聚合 asymmetric selective polymerization不对称立体选择性聚合 asymmetric stereoselective polymerization对映[体]不对称聚合 enantioasymmetric polymerization对映[体]对称聚合 enantiosymmetric polymerization异构化聚合 isomerization polymerization氢转移聚合 hydrogen transfer polymerization基团转移聚合 group transfer polymerization,GTP消除聚合 elimination polymerization模板聚合 matrix polymerization,template polymerization插层聚合 intercalation polymerization无催化聚合 uncatalyzed polymerization开环聚合 ring opening polymerization活性开环聚合 living ring opening polymerization不死的聚合 immortal polymerization酶聚合作用 enzymatic polymerization聚加成反应,逐步加成聚合 (曾用名) polyaddition偶联聚合 coupling polymerization序列聚合 sequential polymerization闪发聚合,俗称暴聚 flash polymerization氧化聚合 oxidative polymerization氧化偶联聚合 oxidative coupling polymerization逐步[增长]聚合 step growth polymerization缩聚反应 condensation polymerization,polycondensation酯交换型聚合 transesterification type polymerization, ester exchange polycondensation自催化缩聚 autocatalytic polycondensation均相聚合 homogeneous polymerization非均相聚合 heterogeneous polymerization相转化聚合 phase inversion polymerization本体聚合 bulk polymerization, mass polymerization固相聚合 solid phase polymerization气相聚合 gaseous polymerization,gas phase polymerization吸附聚合 adsorption polymerization溶液聚合 solution polymerization沉淀聚合 precipitation polymerization淤浆聚合 slurry polymerization悬浮聚合 suspension polymerization反相悬浮聚合 reversed phase suspension polymerization珠状聚合 bead polymerization, pearl polymerization分散聚合 dispersion polymerization反相分散聚合 inverse dispersion polymerization种子聚合 seeding polymerization乳液聚合 emulsion polymerization无乳化剂乳液聚合 emulsifier free emulsion polymerization 反相乳液聚合 inverse emulsion polymerization微乳液聚合 micro emulsion polymerization连续聚合 continuous polymerization半连续聚合 semicontinuous polymerization分批聚合,间歇聚合 batch polymerization原位聚合 in situ polymerization均相缩聚 homopolycondensation活化缩聚 activated polycondensation熔融缩聚 melt phase polycondensation固相缩聚 solid phase polycondensation体型缩聚 three dimensional polycondensation界面聚合 interfacial polymerization界面缩聚 interfacial polycondensation环加成聚合 cycloaddition polymerization环烯聚合 cycloalkene polymerization环硅氧烷聚合 cyclosiloxane polymerization引发剂 initiator引发剂活性 activity of initiator聚合催化剂 polymerization catalyst自由基引发剂 radical initiator偶氮[类]引发剂 azo type initiator2,2′偶氮二异丁腈 2,2'- azobisisobutyronitrile, AIBN过氧化苯甲酰 benzoyl peroxide, BPO过硫酸盐引发剂 persulphate initiator复合引发体系 complex initiation system氧化还原引发剂 redox initiator电荷转移复合物,电荷转移络合物 charge transfer complex, CTC聚合加速剂,聚合促进剂 polymerization accelerator光敏引发剂 photoinitiator双官能引发剂 bifunctional initiator, difunctional initiator三官能引发剂 trifunctional initiator大分子引发剂 macroinitiator引发-转移剂 initiator transfer agent, inifer引发-转移-终止剂 initiator transfer agent terminator, iniferter光引发转移终止剂 photoiniferter热引发转移终止剂 thermoiniferter正离子催化剂 cationic catalyst正离子引发剂 cationic initiator负离子引发剂 ionioic initiator共引发剂 coinitiator烷基锂引发剂 alkyllithium initiator负离子自由基引发剂 anion radical initiator烯醇钠引发剂 alfin initiator齐格勒-纳塔催化剂 Ziegler Natta catalyst过渡金属催化剂 transition metal catalyst双组分催化剂 bicomponent catalyst后过渡金属催化剂 late transition metal catalyst金属络合物催化剂 metal complex catalyst[二]茂金属催化剂 metallocene catalyst甲基铝氧烷 methylaluminoxane, MAOμ氧桥双金属烷氧化物催化剂bimetallic μ-oxo alkoxides catalyst双金属催化剂 bimetallic catalyst桥基茂金属 bridged metallocene限定几何构型茂金属催化剂 constrained geometry metallocene catalyst 均相茂金属催化剂 homogeneous metallocene catalyst链引发 chain initiation热引发 thermal initiation染料敏化光引发 dye sensitized phtoinitiation电荷转移引发 charge transfer initiation诱导期 induction period引发剂效率 initiator efficiency诱导分解 induced decomposition再引发 reinitiation链增长 chain growth, chain propagation增长链端 propagating chain end活性种 reactive species活性中心 active center持续自由基 persistent radical聚合最高温度 ceilling temperature of polymerization链终止 chain termination双分子终止 bimolecular termination初级自由基终止 primary radical termination扩散控制终止 diffusion controlled termination歧化终止 disproportionation termination偶合终止 coupling termination单分子终止 unimolecular termination自发终止 spontaneous termination终止剂 terminator链终止剂 chain terminating agent假终止 pseudotermination自发终止 self termination自由基捕获剂 radical scavenger旋转光闸法 rotating sector method自由基寿命 free radical lifetime凝胶效应 gel effect自动加速效应 autoacceleration effect链转移 chain transfer链转移剂 chain transfer agent尾咬转移 backbitting transfer退化链转移 degradation (degradative) chain transfer加成断裂链转移[反应] addition fragmentation chain transfer 链转移常数 chain transfer constant①缓聚作用②延迟作用 retardation阻聚作用 inhibition缓聚剂 retarder缓聚剂,阻滞剂 retarding agent阻聚剂 inhibitor封端[反应] end capping端基 terminal group聚合动力学 polymerization kinetics聚合热力学 polymerization thermodynamics聚合热 heat of polymerization共聚合[反应] copolymerization二元共聚合 binary copolymerization三元共聚合 ternary copolymerization竞聚率 reactivity ratio自由基共聚合 radical copolymerization离子共聚合 ionic copolymerization无规共聚合 random copolymerization理想共聚合 ideal copolymerization交替共聚合 alternating copolymerization恒[组]分共聚合 azeotropic copolymerization 接枝共聚合 graft copolymerization嵌段共聚合 block copolymerization开环共聚合 ring opening copolymerization共聚合方程 copolymerization equation共缩聚 copolycondensation逐步共聚合 step copolymerization同种增长 homopropagation自增长 self propagation交叉增长 cross propagation前末端基效应 penultimate effect交叉终止 cross terminationQ值 Q valuee值 e valueQ,e概念 Q, e scheme序列长度分布 sequence length distribution侧基反应 reaction of pendant group扩链剂,链增长剂 chain extender交联 crosslinking化学交联 chemical crosslinking自交联 self crosslinking光交联 photocrosslinking交联度 degree of crosslinking硫化 vulcanization固化 curing硫[黄]硫化 sulfur vulcanization促进硫化 accelerated sulfur vulcanization过氧化物交联 peroxide crosslinking无规交联 random crosslinking交联密度 crosslinking density交联指数 crosslinking index解聚 depolymerization①降解②退化 degradation链断裂 chain breaking解聚酶 depolymerase细菌降解 bacterial degradation生物降解 biodegradation化学降解 chemical degradation辐射降解 radiation degradation断链降解 chain scission degradation自由基链降解 free radical chain degradation无规降解 random degradation水解降解 hydrolytic degradation热降解 thermal degradation热氧化降解 thermal oxidative degradation光降解 photodegradation光氧化降解 photo oxidative degradation力化学降解 mechanochemical degradation接枝聚合 graft polymerization活化接枝 activation grafting接枝点 grafting site链支化 chain branching支化度 degree of branching接枝效率 efficiency of grafting接枝度 grafting degree辐射诱导接枝 radiation induced grafting嵌段聚合 block polymerization---------------------------通用类:高分子 macromolecule, polymer超高分子 supra polymer天然高分子 natural polymer无机高分子 inorganic polymer有机高分子 organic polymer无机-有机高分子 inorganic organic polymer金属有机聚合物 organometallic polymer元素高分子 element polymer高聚物 high polymer聚合物 polymer低聚物 oligomer二聚体 dimer三聚体 trimer调聚物 telomer预聚物 prepolymer均聚物 homopolymer无规聚合物 random polymer无规卷曲聚合物 random coiling polymer头-头聚合物 head-to-head polymer头-尾聚合物 head-to-tail polymer尾-尾聚合物 tail-to-tail polymer反式有规聚合物 transtactic polymer。
冶金原理之塑性变形与回复再结晶
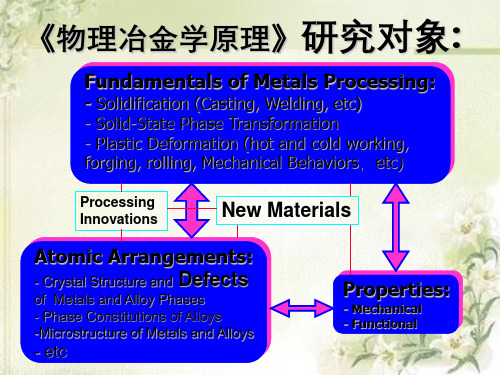
有一个滑移方 向<111>!
滑移面滑移方向上的分切应力
Resolved Shear Stress on Slip Direction
= s cosl cosf = m.s
Schmidt 因子
Resolved Shear Stress on Slip Direction
Basic Modes of Plastic Deformation for Metallic Single-Crystals
滑移 Slip 孪晶 Twining
滑移 Slip
在切应力作用下晶体沿特定晶面上特 定晶向产生相对平移滑动的现象
滑移现象
滑移的本质及滑移系
滑移变形的特点
滑移Slip
在切应力作用 下,晶体沿特 定晶面上特定 晶向产生相对 平移滑动的塑 性变形现象
ss se
d
Strain, %
金属的典型拉伸(应力-应变)曲线
Typical Tensile Curves of Metallic Materials
Stress, MPa
sb
ss se
d
Strain, %
弹性变形
Elastic Deformation:
塑性变形
Plastic Deformation:
• 不同晶粒位向不同,变形不同时性与不均匀 性
• The Role of GBs in Plastic Deformation at RT and High Temperatures
• The Important Role of GBs to Strength (Hall-Petch Equation) and Plasticity of Metals
废旧聚乙烯催化降解制备聚乙烯蜡_张建雨
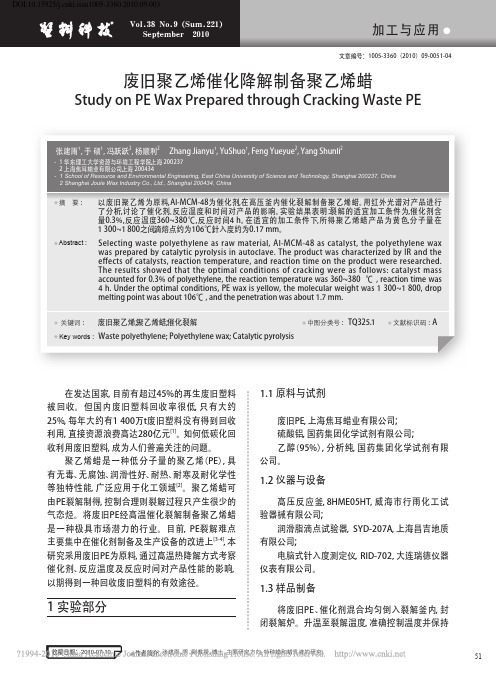
收稿日期:512010-07-10废旧聚乙烯催化降解制备聚乙烯蜡Study on PE Wax Prepared through Cracking Waste PE作者简介:张建雨,男,副教授,博士,主要研究方向:特种蜡和蜡乳液的研究。
张建雨1,于 硕1,冯跃跃2,杨顺利2 Zhang Jianyu 1, YuShuo 1, Feng Yueyue 2, Yang Shunli 2- 1 华东理工大学资源与环境工程学院,上海 200237 2 上海焦耳蜡业有限公司,上海 200434- 1 School of Resource and Environmental Engineering, East China University of Science and Technology, Shanghai 200237, China 2 Shanghai Joule Wax Industry Co., Ltd., Shanghai 200434, China摘 要 :以废旧聚乙烯为原料,Al-MCM-48为催化剂,在高压釜内催化裂解制备聚乙烯蜡。
用红外光谱对产品进行了分析,讨论了催化剂、反应温度和时间对产品的影响。
实验结果表明:裂解的适宜加工条件为,催化剂含量0.3%,反应温度360~380℃,反应时间4 h。
在适宜的加工条件下,所得聚乙烯蜡产品为黄色,分子量在1 300~1 800之间,滴熔点约为106℃,针入度约为0.17 mm。
Abstract :Selecting waste polyethylene as raw material, Al-MCM-48 as catalyst, the polyethylene wax was prepared by catalytic pyrolysis in autoclave. The product was characterized by IR and the effects of catalysts, reaction temperature, and reaction time on the product were researched. The results showed that the optimal conditions of cracking were as follows: catalyst mass accounted for 0.3% of polyethylene, the reaction temperature was 360~380℃, reaction time was 4 h. Under the optimal conditions, PE wax is yellow, the molecular weight was 1 300~1 800, drop melting point was about 106℃, and the penetration was about 1.7 mm. 关键词 :废旧聚乙烯;聚乙烯蜡;催化裂解Key words : Waste polyethylene; Polyethylene wax; Catalytic pyrolysis文章编号:1005-3360(2010)09-0051-04在发达国家,目前有超过45%的再生废旧塑料被回收。
- 1、下载文档前请自行甄别文档内容的完整性,平台不提供额外的编辑、内容补充、找答案等附加服务。
- 2、"仅部分预览"的文档,不可在线预览部分如存在完整性等问题,可反馈申请退款(可完整预览的文档不适用该条件!)。
- 3、如文档侵犯您的权益,请联系客服反馈,我们会尽快为您处理(人工客服工作时间:9:00-18:30)。
a r X i v :0707.4014v 2 [c o n d -m a t .s o f t ] 13 S e p 2007Reversible plasticity in amorphous materialsMicah Lundberg 1,Kapilanjan Krishan 1,Ning Xu 2,3,Corey S.O’Hern 4,5and Michael Dennin 11Department of Physics and Astronomy,University of California at Irvine,Irvine,CA 92697-45752Department of Physics and Astronomy,University of Pennsylvania,Philadelphia,PA 19104-63963James Franck Institute,The University of Chicago,Chicago,IL 606374Department of Mechanical Engineering,Yale University,New Haven,CT 06520-82865Department of Physics,Yale University,New Haven,CT 06520-8120(Dated:February 1,2008)A fundamental assumption in our understanding of material rheology is that when microscopic deformations are reversible,the material responds elastically to external loads.Plasticity,i.e.dis-sipative and irreversible macroscopic changes in a material,is assumed to be the consequence of irreversible microscopic events.Here we show direct evidence for reversible plastic events at the microscopic scale in both experiments and simulations of two-dimensional foam.In the simula-tions,we demonstrate a link between reversible plastic rearrangement events and pathways in the potential energy landscape of the system.These findings represent a fundamental change in our understanding of materials—microscopic reversibility does not necessarily imply elasticity.PACS numbers:05.20.Gg,05.70.Ln,83.80.IzOne of the fundamental questions in materials sci-ence concerns the microscopic origin of plastic behavior.Why do materials display plastic rather than elastic and reversible response and can we predict for what loads this will occur?An improved understanding of plastic deformation is especially important in a wide range of amorphous materials,such as metallic[1,2]and poly-meric glasses[3],viscoplastic solids[4],foams[5],granular materials[6],colloids[7,8],emulsions[9],and even intra-cellular networks[10].In crystalline materials,plastic be-havior is understood in terms of defect nucleation and dynamics[11,12].However,for amorphous materials,a description in terms of topological defects is not possible due to inherent structural disorder.Therefore,identify-ing and characterizing local plastic events in amorphous materials is essential for a complete understanding of their structural and mechanical properties.The conven-tional wisdom is that plastic rearrangement events cause irreversible structural changes in these materials on the microscale.The macroscopic response of amorphous solids and complex fluids,such as foams,colloids,and granular matter,to applied stress and strain is very similar:elastic at small strains and plastic at larger strains.In the elastic regime,stress is proportional to applied strain,and deformations are reversible.Above the yield stress or strain,plastic flow or anelastic deformation oc-curs.Given these similarities on the macroscopic scale,many models of plasticity have emphasized the impor-tance of microscopic “plastic zones”within amorphous materials[2,13,14,15,16,17,18]in which neighbor switching and other rearrangements events of “particles”occur.(The particles represent molecules in the case of solids,or bubbles or grains in the case of complex flu-ids.)An important open question concerning plastic-ity is whether or not plastic zones are intrinsically ir-reversible or,instead,is their surrounding environment ultimately responsible for determining whether or not re-arrangement events are reversible?We perform both experiments and simulations of two-dimensional amorphous foams undergoing oscillatory shear strain to investigate this fundamental question.In both cases,we find a significant fraction of dissipative,plastic rearrangement events that are reversible ,even for strains significantly above the yield strain.In the simula-tions,measurements of the local potential energy allows us to assess the impact of the bubble’s neighborhood on the reversibility of the plastic events.This links reversible plastic rearrangement events to pathways in the poten-tial energy landscape of the system during deformation.We argue that even during plastic flow certain micro-scopic rearrangement events are intrinsically reversible and changes in the environment surrounding plastic zones determine whether the zones are reversible or not.We chose bubble rafts[4,19,20,21]that consist of gas bubbles floating on a water surface for our experimen-tal system.For the simulations,we employed the well-characterized bubble model for two-dimensional (2D)foams developed by Durian[22].The bubble model as-sumes massless circular bubbles that interact through a repulsive linear spring force and viscous dissipation.Experimental evidence supports the applicability of the bubble model to explain the flow behavior of bubble rafts,as well as three-dimensional foam[21,23,24].Even though other rearrangement events occur in bubble rafts and the bubble model,plastic rearrangement events known as T1events play a central role in the mechanical response of foam[20,21,25,26,27,28,29,30,31].T1events correspond to a neighbor switching event in which two neighboring bubbles lose contact,and two next-nearest neighbors become neighbors[5].This cor-responds to a transition between two distinct states of the system.For example,referring to Fig.1,State A is when bubbles 1and 2are neighbors,and State B is when2bubbles3and4are neighbors.For both the experiment and the simulations,during one cycle the applied shear strain varies from0to A/L y(at phaseψ=π)and back to a strain of0(atψ=2π),where A is the amplitude of the shear displacement and L y is the system size in the shear-gradient direction.If four bubbles experience a T1event that switched the bubbles from state A to B during thefirst half-cycle of the drive,a reversible T1 event occurs if the same foursome of bubbles returns to state A in the second half-cycle of the drive.Otherwise, the T1event is irreversible.For the experiments,the system contained approxi-mately800bubbles in a planar shear cell with L y=9cm. Half of the bubbles were2mm in diameter and the other half were3.5mm.We report on results using driving am-plitudes A of10and12times the diameter of the small bubbles and driving frequency0.2s−1.The resulting rms strain and strain rate were approximately0.2and 0.04s−1,respectively.This should be compared with the yield strain of0.01for bubble rafts and thetransition to quasi-static behavior on the order of0.07s−1[32].At the yield strain,T1events give rise to permanent plastic deformation[33].Details of the experimental setup for bubble rafts can be found in Ref.[34].In the bubble model simulations,bidisperse systems composed of N/2large and N/2small circular bubbles with diameter ratio r=1.75were used to match exper-iments.We studied square simulation cells with system sizes in the range N=16to1024and packing fraction φ=0.95,so that the bubbles were always compressed during shear.The bubble model treats foams as mass-less deformable disks with an equation of motion that balances a linear repulsive spring force to model elas-tic repulsion with viscous dissipation proportional to lo-cal velocity differences[22].The oscillatory shear strain is applied quasistatically to the system by shifting the x-positions of the bubbles,implementing shear-periodic Lees-Edwards boundary conditions[35]and minimizing the total potential energy.To study the role of the en-ergy landscape,two definitions of the local potential en-ergy based on the overlaps between bubbles were used,E and E′.E is computed only considering overlaps among the four bubbles defining the T1event,while E′also in-cludes overlaps with thefirst nearest neighbors of the T1 bubbles.Finally,we measured∆E′,defined by subtract-ing the potential energy E′of the four bubbles partici-pating in the reversible T1event from the original oscil-latory shear strain simulations with E′from simulations in which the four T1bubbles are forced to exactly re-trace their positions as they transition from state B back to state A,but all other particles are allowed to move without constraints.To fully understand the behavior of the system,it is best to directly compare the reversible and irreversible rearrangement events from both experiments and simu-lation.Panels(a)and(b)of Fig.1(experiment)and FIG.1:(color online)Experimental results for a reversible [(a)and(b)]and an irreversible T1event[(c)and(d)].The images in(a)and(c)are10.5mm×10.5mm with bubbles in-volved in the T1events labeled by numbers.The roman label for each image in(a)and(c)corresponds to the same label on the trajectory in(b)and(d).(a)The images highlight the following stages of the reversible T1event:initial state (i),middle of the T1event(ii),second state(iii),middle of the T1event under reversal of shear(iv),and the return to the initial state(v)[36].(b)Plot of the trajectory of bubble1 in the images in(a).Two consecutive cycles are shown(the first in black squares and the second in red circles).(c)The images highlight the following stages of the irreversible T1 event:initial state(i-iii),middle of the T1event(iv),and the second state(v-vii).(d)Plot of the trajectory of bubble 4in the images in(c).Three consecutive cycles are shown (thefirst in black,the second in red,the third in green). Fig.2(simulation)highlight typical reversible T1events. Panels(c)and(d)of Fig.1(experiment)and Fig.2(sim-ulation)highlight irreversible events.The plots focus on the four bubbles(labeled1-4)that experience a T1 event.Snapshots are used to illustrate bubble motions during a typical T1event(panels(a)and(c)).For the experiments,panel(b)and(d)highlight the trajectory ofa single bubble in real space.For the simulations,panels(b)and(d)display the potential energy E as a function of the phase of the driving.The simulation results are for N=16,but similar results were obtained with much larger systems with N=1024.The defining feature of reversible T1events is that the initial andfinal states of the bubbles in the T1event are equivalent,despite the occurrence of dissipation.The dissipation is evident in the anharmonic behavior of the local potential energy signal.This is in contrast to the perfectly elastic behavior for small shear strains in the absence of T1events shown in the inset to Fig.2b.The existence of the dissipation leads to a number of asym-metries in the dynamics of the system,despite the overall periodic nature of the response.The spatial trajectory3FIG.2:Results taken from a16-particle simulation of the bubble model in two dimensions undergoing oscillatory shear strain with an amplitude of2small bubble diameters for a reversible[(a)and(b)]and an irreversible event[(c)and(d)]. Bubbles involved in the T1events are labeled by numbers. Roman labels in the images correspond to the same labels in the plots.(a)Images(i)-(iii)show the occurrence of a re-versible T1event and(iv)and(v)show the reversal of the T1event.(See the supplementary information for a movie of this event.)(b)The local potential energy E(solid black line)is plotted versus the driving phase(left axis).For com-parison,the periodic strain is plotted with a long dashed blue line(right axis).The elapsed phase for the T1event is sig-nificantly different than that for the reversed T1event,and the shape of the local potential energy is not the same for the T1event and its reverse.The inset shows an elastic response in E vsψ/πfor small amplitude oscillations A=10−2times the small bubble diameter,where the response matches the driving.(c)Images(i)-(iii)show the occurrence of an irre-versible T1event,as shown by the absence of the reverse T1 event in images(iv)and(v)[36].(d)The local potential en-ergy E(solid black line)is plotted versus the driving phase (left axis).The periodic strain is plotted with a long dashed blue line(right axis).Note that E for locations(i)and(v) separated by a phase interval of2πare not the same.is a closed loop with afinite area(see Fig.1b).This causes the symmetric rearrangements of the four bubbles during the two T1events(images(ii)and(iv)in Fig.1a) to occur at different locations during the corresponding half-cycle.Likewise,Fig.2b illustrates that E is very different for the two half cycles corresponding to labels (i)-(iii)and(iv)-(v).Finally,the durations of the T1 event and its reverse(state A to B vs.state B to A)are not the same.During irreversible T1events,the defining feature is that a foursome of bubbles undergoes a T1event in the first half cycle of the driving but the reverse T1event does not occur during the second half cycle.The exper-imental example in Fig.1c is a case where a single T1 event from state A to B occurred during seven cycles of the driving(three of which are highlighted in Fig.1c). The impact of the T1event on the trajectories in Fig.1d is dramatic.The trajectory of bubble four is shown for00.20.40.60.81ψ/π0.050.10.15<∆E’>−0.100.10.20.30.4∆E’10203040P(∆E’)00.20.40.60.81ψ/π−0.10.10.20.3∆E’(a)(b)FIG.3:(a)The local potential energy difference ∆E′ av-eraged over100reversible T1events plotted vs.the driving phase under the same conditions in Fig.2. ∆E′ >0con-firms that exact trajectory reversal is not energetically favor-able.The inset shows∆E′for a single reversible T1event.(b)The probability offinding a particular∆E′.There is a large peak at∆E′=0,two slight peaks near0.15and0.30, and no significant weight in the distribution for∆E′<0. three cycles:just before the T1event(black),during the T1event(red),and just after the T1event(green).In the absence of a T1event,the local trajectory essentially repeats itself during each half-cycle as the bubbles move along similar paths.The occurrence of the T1event rep-resents a dramatic break in this motion.The behavior of irreversible T1events in simulations is similar to that found in experiments.Figure2c illustrates a T1event that occurs during frames(i)-(iii)in thefirst half cycle,but the T1event is not reversed during the second half cycle in frames(iii)-(v).The plot of E in Fig.2(d)illustrates that configurations(i)and(v),which are separated by2πin phase,do not have the same local potential energy.Note that beyondψ/π∼5.5,the local potential energy signal is periodic,which indicates that other T1events or possibly more complex rearrangement events that occur in the system are reversible.What is the connection between reversible T1events and the path that the system follows through the poten-tial energy landscape?Answering this question provides initial insights into why some T1events are reversible and others are irreversible and how the system returns to the same potential energy minimum even though it follows a different path in the energy landscape during the T1 event and its reverse.The calculation of∆E′obtained by comparing the local potential energy E′(including inter-actions of T1bubbles withfirst nearest neighbors)of the four bubbles in the original oscillatory shear strain simu-lations with E′from the constrained simulations directly addresses this question.The results from these studies are shown in Fig.3. First,in Fig.3(a)wefind that the local potential energy4 difference averaged over many T1events from indepen-dent runs ∆E′ >0.∆E′for a single T1event shownin the inset to Fig.5(a)has large positive spikes but also significant phase intervals where∆E′=0.In Fig.3(b), we show that the distribution P(∆E′)of energy differ-ences has a strong peak at zero,but non-negligible peaks at∆E′≈0.15and0.3and no significant weight for ∆E′<0.Each of thesefindings indicates that the path during the second half cycle that does not exactly retrace the path in configuration space of thefirst half cycle is energetically favorable.Furthermore,for the case of re-versible T1events,it is likely that there are a number of local low energy pathways that lead from state B back to state A.For the case of irreversible T1events,it is likely that there are many local energy pathways away from state B,but the ones that are energetically favor-able do not lead back to state A.Since our system is athermal,these differences in the energy pathways are due to changes in the environment(surrounding bubbles) that occur during the applied shear strain.Thus,we ar-gue that the influence of the environment gives rise to the irreversibility of T1events in foams.In equilibrium systems,thermalfluctuations will also play a significant role in determining reversibility.Our experiments and simulations of model foams un-dergoing oscillatory shear strain identify reversible T1 events,which are two state systems.This observation is thefirst direct experimental confirmation of a general two-state model of plasticity:shear transformation zones (STZ).The concept of a STZ as a reversible,two-state transition within a material wasfirst proposed by Falk and Langer[14].The STZ picture is successful in explain-ing a range of macroscopic behavior of materials based on dynamics of the microstructure.STZ’s represent a natural extension of ideas based on activated transitions and free volume[1,37,38]and it has motivated a num-ber of other models of plasticity[18,39,40].Therefore, our results establish the applicability of two-state STZ models to athermal particulate systems,and the need to include intrinsically reversible plastic events in models of plasticity.Our studies of the local potential energy landscape go beyond the two-state model and establish the importance of the accessible pathways in the energy landscape that ultimately determine the reversibility of the plastic events.Thus,we have learned that plastic-ity does not imply microscopic irreversibility and that microscopic reversibility does not imply elasticity. Financial support from the Department of Energy grant numbers DE-FG02-03ED46071(MD),DE-FG02-05ER46199(NX),and DE-FG02-03ER46088(NX),NSF grant numbers DMR-0448838(CSO,GL)and CBET-0625149(CSO),and the Institute for Complex Adaptive Matter(KK)is gratefully acknowledged.We also thank M.Falk for insightful conversations.[1]A.S.Argon,Acta Metallurgica27,47(1979).[2]M.L.Falk,nger,and L.Pechenik,Physical Re-view E70,011507(2004).[3]M.D.Ediger,Annual Review Of Physical Chemistry51,99(2000).[4]L.Bragg,Journal of Scientific Instruments19,148(1942).[5]D.Weaire and S.Hutzler,The Physics of Foams(Claren-don Press,Oxford,1999).[6]C.H.Liu and S.R.Nagel,Phys.Rev.B48,15646(1993).[7]E.R.Weeks,J.C.Crocker,A.C.Levitt,A.Schofield,and D.A.Weitz,Science287,627(2000).[8]E.R.Weeks and D.A.Weitz,Physical Review Letters89,095704(2002).[9]T.G.Mason,J.Bibette,and D.A.Weitz,Journal ofColloid and Interface Science179,439(1996).[10]D.A.Head,A.J.Levine,and F.C.MacKintosh,PhysicalReview E68,061907(2003).[11]J.R.Rice and R.Thomson,Philosophical Magazine29,73(1974).[12]J.R.Rice,Journal Of The Mechanics And Physics OfSolids40,239(1992).[13]J.C.Baret,D.Vandembroucq,and S.Roux,PhysicalReview Letters89,195506(2002).[14]M.L.Falk and nger,Physical Review E57,7192(1998).[15]M.Heggen,F.Spaepen,and M.Feuerbacher,Journal OfApplied Physics97,033506(2005).[16]nger,Physical Review E73,041504(2006).[17]A.Onuki,Physical Review E68,061502(2003).[18]G.Picard,A.Ajdari,L.Bocquet,and F.Lequeux,Phys-ical Review E66,051501(2002).[19]A.S.Argon and H.Y.Kuo,Materials Science And En-gineering39,101(1979).[20]A.Abdel Kader and J.C.Earnshaw,Physical ReviewLetters82,2610(1999).[21]M.Dennin,Physical Review E70,041406(2004).[22]D.J.Durian,Phys.Rev.Lett.75,4780(1995).[23]uridsen,M.Twardos,and M.Dennin,Physical Re-view Letters89,098303(2002).[24]A.D.Gopal and D.J.Durian,Physical Review Letters75,2610(1995).[25]Y.Wang,K.Krishan,and M.Dennin,PhilosophicalMagazine Letters87,125(2007).[26]M.Dennin and C.M.Knobler,Physical Review Letters78,2485(1997).[27]B.Dollet,M.Durth,and F.Graner,Physical Review E73,061404(2006).[28]D.A.Reinelt and A.M.Kraynik,J.Rheol.(N.Y.)44,453(2000).[29]M.F.Vaz and S.J.Cox,Philosophical Magazine Letters85,415(2005).[30]D.Weaire,F.Bolton,T.Herdtle,and H.Aref,Phil.Mag.Lett.66,293(1992).[31]S.Vincent-Bonnieu,R.H.Hohler,and S.Cohen-Addad,Europhysics Letters74,533(2006).[32]E.Pratt and M.Dennin,Physical Review E67,051402(2003).[33]S.Hutzler,Ph.D.thesis,Trinity College,Dublin(1997).[34]M.Lundberg,K.Krishan,,N.Xu,C.S.O’Hern,andM.Dennin,in preparation(2007).5 [35]M.P.Allen and D.J.Tildesley,Computer Simulation ofLiquids(Oxford University Press,Oxford,1987).[36]See EPAPS Document No.[]for movies of the reversible(ExperimentReversible.mov,SimulationReversible.mov)and irreversible(ExperimentIrreversible.mov,Simula-tionIrreversible.mov)events from both the experimentsand simulation.For more information on EPAPS,see/pubservs/epaps.html.[37]V. A.Khonik and A.T.Kosilov,Journal Of Non-Crystalline Solids170,270(1994).[38]F.Spaepen,Acta Metallurgica25,407(1977).[39]L.Berthier,Journal Of Physics-Condensed Matter15,S933(2003).[40]G.Picard,A.Ajdari,F.Lequeux,and L.Bocquet,Eu-ropean Physical Journal E15,371(2004).。