A brief introduction to Organocatalysis
文献综述报告范例
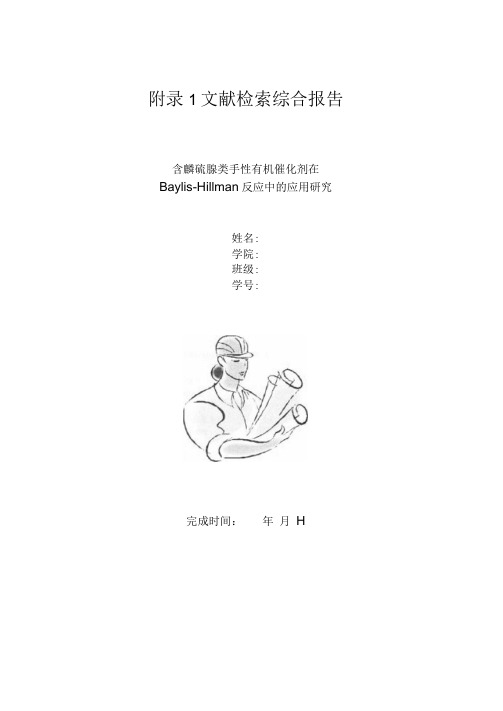
附录1文献检索综合报吿含麟硫腺类手性有机催化剂在Baylis-Hillman反应中的应用研究姓名:学院:班级:学号:完成时间:年月H282 I 文献检索与知识发视悟南3.松供步骤及检寧结火 .................................................... 2RIt 1履件中更科技期阿戟盘13.2中ININ 家知Ul 产权虻数据障 ........ — ............................. …郵 X3 CA 网络扳散皿库 ................................................... 245 1 4 •利 .......................................... — ............... 2» 3. 5 SCI 散鹏除 ......................................................... 加 L6 Enuuiecnng Villa^i (EbftlbiH ................... ...................................................... •• 288 5•啊内外研吨槪况1.讹聴分析 X 4倉策昭2. 1柠啾 X 2卄通川JP6:JPEG 图像283 283 2&3 2832W素像61A6加录 1 文I 2831•课题分析Th “llmxm 反庄足•矣妙命右炮用确就的令机合成尺弘它卑存催化fKMUWK. 怜)作用卜需件怖'Z.flH 的反*砒列 CH 血多官能团的户材.液反应条件汨和MH 召 口好的14"鏡济性和述仟件•卩I 足 八 連度愷•产和I 〔•以效彫响I 『处合fiU.的颐・为「黒岛反心堆臣.人们 片A 伤力,找存仗的催化刑•卅用的俗化卅包括般炽“ULG 仁 勺斗矣催化笊押比"汕1・亲檜性吏舉•从廉反应的忆应机现来依冇机聃圧俊反丈更 竹效的侪化)Me«WM 含・机催化刊•试类依化刑fL Baylis Hillman 反 ^juiujRw^允分的mr.w4t 対找代絞晝合段的y 性化方法御男覆大的拓巌勺 甸化.本*峻斤e 利用这学開所学的又献稅爲如识•檢痢E 内外竹关:tuflwi 那夬F-nn FI 借化UftBaylto KillrnnfigtH 为文家 MWJiTj 整理•时国内外杠“MUMF 竹仔机催化WARnyhs Hdlnumfii^中的应用方血研究的域霸进廉仆一定的「解•通M 对研克进雇的分析• 了解含・凑■矣「件柿机修化刑用十KAylis Hillman X 心所“$的问 越•从向思号解决何题•貰為儿催化沾性与迅捋竹的方出.2.1检索工具的选择iiJii 一个化学&屯柯关谭觀.故必检数聚申如**(1>中M 专利数据H«19R5至今》 聲曹中文WJHtt®^<1989至令)<2>外文敷IK 斤:矣国化爭心(网恪版• 1907至令〉 歐洲矽利同致祈申1975至今)SCI1985 玄今》l :W1t-Jitt«f4-<l98O 至今》 2.2检索词的选择的选杼l£注倉树义诃庞文诃•不同拼“方式筑<!)中文ItKMi槓心檢磺词'M.aW.BaylitHdlnumII 他检*竹机催化制■ F 件翔化制出HM 映选择性•不甘称仏携宛洙越的初期•主戏说用谀题的孩心诃做込勺的组配成为怡欢成进荷文鍬令找<:♦ t WK3MUUU 僧征的IWBvU Mi —w ; i 咖MHW 中同的2.检5像66 S 7 GEKBX &IPE0446 p J 1 5 •J -2型小才QQ类大尺・• 《址仃4制・"计公花厦桃火的出检•m"的存全申・所口场曲杞诫詞处斤刃 0ttayl» liiUman- 「旳|東进, •鸽■負鼻伞*.血轲十檢PWTfffl ■化auis 检*馆况・金0鞍对“”拆分册此 刃外・tn 耳也审克屮讯如尺用•研克之更灼呵 文A ■吃的舛豪&役K 为同•不炖咀公全*.所以・於:1斥析•低时•宣KaylK Hdlmam ■•箕諒汀件・仃现催ft :狎力•的中文•化检伏讨用中直IWttGlS 的旳 4ILH 和ki4tuufiMHftr (mft ■•庄保M 金令卒的mat 卜■囲令用•・<:>英文檢ft 剑,代心检*Sh phcwphinDthKMrai«phu ;«phmr> thsMirm jheounn |4io*phinr Jteyhn IliUfiMn.H II fT4KtH»UX Ife 1^ W Ml chind. chirality* ur|pmor»ulyM % orsMoratiilyv. rnuntkwicrtmty. mant iQHclcctiw ^aaymnwinc的号世梨此皿衣河的农”彫式・使用戲诃符<4以M 人条MT •良奔杭*第*金13诵用检索式z| W •檢触• lUtyl < Hiihtuio B H 反 •彳川样 • 2・•““3・・ (Huylu Hiltmiin 4 不H 你“对喰連昂,I MW -<Hsyi^ Iblbnan t对样・ 4心托)0 5 bayli» Hilhian ♦ H H 只依《水耳你卜0 A |4Mi^|*hifn>th«Mirrn •< |»hfx|4ufio ilMounn 4 thunirv/i |iho<phinfn 7 Baylis Hillmn + B H rramuti ♦ ”ymmrtnt 4 chinl + cmniw»rkcfi%T 也屮•••■衣小厦糾-‘广,・4欢检當结》!«41皿 詢".3.检索步鼻及检索结果3.1缁醫中文料技期利敷期■检*式二M ・・・(知^Uylis Wlbwm+Wh2 B 文献•MmiuN(1) [9& K] Ttl •分于借化的从&yh ・ Hillrwm 阪Q 产审枷甲星U ・舅iMM 种1建化—Mt 内OBMl 倫“ XI X im 恂1不特I 文献桧第塚合报告.285(N 名】右机化学・2008・28(8八1「・(町1500 (ISSN 号】0253 • 2786m-chird • -fl 为*“• «!M 的审丸II 的.吐??为•卿*A 达〃式.IWUIHIA HI 中的般分flivmiA 检水式•以(关键同】丁埔内酯術生詢•:屮*灯氓选择性反应产物处瞇催化小物活性化合物【分类号】O62L6TQ655【文摘】(略)⑵【物名】瞬储化的Baylis Hdhnan反应【作K )裡册雷王挣果秀故刘谢燕冯涪【机构】河北农业大学理子院•保定07IIMH(f<j 名)右机化学<2008.28(2>: 191-200(ISSN 兮】O253-278G【关確同】Bayli^Hillfiuin反应活性烯叔瞬催化剣【分类号】()621.3 TQ0I5.9【文摘)(略)为了全面了昭Hayliz HiUnuut反应的硏丸•对増加检索式M=Riy矗Hillman•命屮27 篇文献.乂选中-・简综述性丈飲如F >(3> 名】IbyliLHillman反応机用研丸进展【作不】杨祚紅樊建芬乔龙尢【机构】苏州人学化学化工学院•苏州215123(W 名】右机化学.2OO8.2B(2): J75* 180(ISSN 号】0253 - 2786【关谜词)BhyliHlillmmi反应反应机理综述〔分<)643.120621.3【文和(略)3.2中国国家知识产权局数据廉檢険式:命中一第检索结果・(4)中请号(200610118184.7 中请th 2006. 11,10名称’化仟物•合成片法及助応用公开(公吿)V: CN1948320 公开(公^)H, 2007.04. 18上分类I矢C07E9/50(2006.01)l 分案原巾请号,分类叭C07F9/5D(2006. 01 > b CO7C22!/00 < 2006. 01) I;('07( 725 6 (2006. 01>l;WHJ31/ 02(2006.01)1申请心利权〉人:中国科学院I:海有机化学研处斫地址:200032 I•酶市徐汇区枫林路354号发明(址卄)人*时水殃•他玻国际阳询:国际公布,进人IH家II期:擒耍:(峪)3.3 CA网络版数据库检索式:l)hcW>HwxhkHiira uK(ph^Juix)iluuurci or Huouw pliosphinc)and B(iylt5 Hillman)<5; Chiral phosphinothiourea organocatalyst In the enantioselective Morita- Baylis-Hillmanreaction ・ of aromatic aldohydes with methyl vinyl ketone«、Sm Kuu ZhAnx« Ixi; Sonu« Han< I Yinjuni Wu> Xin- Y r an. I JI I> lor AdvarxttlMiiirruds and InMitutc uf F IIH ChmMcals. E A ・I Chinn I niv< rMty of N H nn anti I'cchnoloKy* ShamKluiu Pmp.6264. F^blishtTt Elsevier Ltdin HnglisK CAN 149: 536239Abstract :(略) (6) Chiral thiourea-phosphine organocatalysfs in the wymmetric aza-Morit»Bayli^HlIlman roaction ・ Shi. X I ingt Siu. Miru S it< K<> IJit<jnnnry of OrKxnonictnllic ( hcmistry. Shanuhm liishtwir of (Irgnmc Cb<rniMnr>「hinrse A CMC K TOV al Scicrxvs. Skingliai* Prop. R 屮 Chimu Advanced Synth ・ w & ( ntnlysi* ( 3(K )7)•・:■ (13). 2129 2135. Publi4)<r t WihyWH Vrrl^ GmbH ft Co. K(;A A. C()DEN IASCAE7 ISSN, 1615 - 1150. Joumnl wntt<n in 臥14 CAN 119; 3, 73<5 AN - o7: 1114581CAPLUSAbstract :(7) Pro8ss for preparation of thiourea substituted chiral BINAPs as asymmetric catalysts for Baylis-Hillman reaction ・ Shi. VonghnKi Shu Mm. (Shangka hziiuwuf (>rg^ni (( heniiMry • Chim M Aouh my of K irnce^. Pcopu R<、p ・ China >. Himnu* Zh ・:mli S1)rn<|ini : < vongkai Sliuonung^hu ( 2007 ) • 1 pp. (()[>E\| CXXXEV I N l!Hb3?n \ 2^07()418 Potent written in l^hirwAC« Applicationg2006 101181 H I 20061110. PriorityiCAN 1I6I 501180 AN 2007, 436241 Abstract*(K) Cooperative t Highly Enantloeelective Phosphinothiourea Catalysis of Imine-Allene [3 令 2] Cycloaddition 。
抗生素概论(英文PPT) A brief review of antibiotics
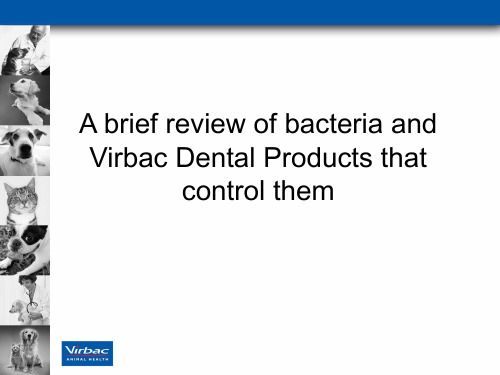
• Germicide-agent that kills pathogenic microorganisms
• Bacteriostatic
– Stops the bacteria from multiplying but does not kill it
• Bactericidal
– Kills the bacteria
– Examples
• Some work by interfering with protein synthesis • Some work by interfering with cell wall function • Some work by interfering with bacterial DNA
M acintosh PIC T im age form at
• Resistance- bacteria are no longer susceptible to the agent
Classes of Antimicrobials
• Classified based on general spectrum and how they work and how they are made
• Shape of bacteria
– Cocci- circle shaped
– Rods- cylindrical
Terminology
• Antimicrobial
– Any substance of natural, semi-synthetic, or synthetic origin that kills or inhibits the growth of a microorganism, but causes little or no host damage
2021 年度诺贝尔化学奖:大道至简
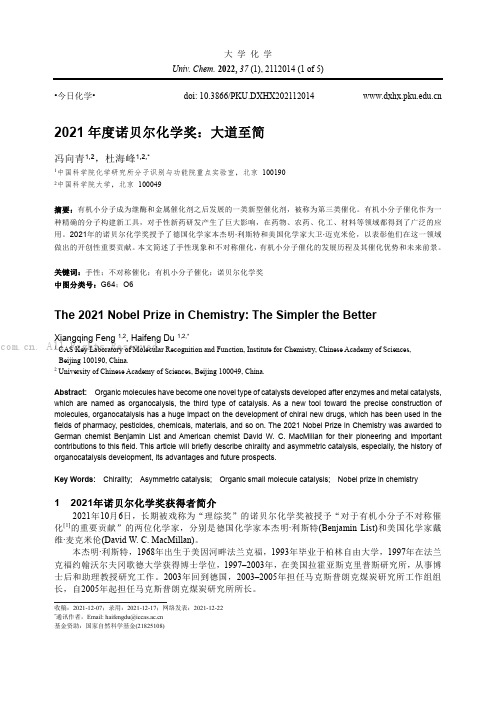
大 学 化 学Univ. Chem. 2022, 37 (1), 2112014 (1 of 5)收稿:2021-12-07;录用:2021-12-17;网络发表:2021-12-22*通讯作者,Email:******************.cn基金资助:国家自然科学基金(21825108)•今日化学• doi: 10.3866/PKU.DXHX202112014 2021年度诺贝尔化学奖:大道至简冯向青1,2,杜海峰1,2,*1中国科学院化学研究所分子识别与功能院重点实验室,北京 1001902中国科学院大学,北京 100049摘要:有机小分子成为继酶和金属催化剂之后发展的一类新型催化剂,被称为第三类催化。
有机小分子催化作为一种精确的分子构建新工具,对手性新药研发产生了巨大影响,在药物、农药、化工、材料等领域都得到了广泛的应用。
2021年的诺贝尔化学奖授予了德国化学家本杰明·利斯特和美国化学家大卫·迈克米伦,以表彰他们在这一领域做出的开创性重要贡献。
本文简述了手性现象和不对称催化,有机小分子催化的发展历程及其催化优势和未来前景。
关键词:手性;不对称催化;有机小分子催化;诺贝尔化学奖中图分类号:G64;O6The 2021 Nobel Prize in Chemistry: The Simpler the BetterXiangqing Feng 1,2, Haifeng Du 1,2,*1 CAS Key Laboratory of Molecular Recognition and Function, Institute for Chemistry, Chinese Academy of Sciences, Beijing 100190, China.2 University of Chinese Academy of Sciences, Beijing 100049, China.Abstract: Organic molecules have become one novel type of catalysts developed after enzymes and metal catalysts, which are named as organocalysis, the third type of catalysis. As a new tool toward the precise construction of molecules, organocatalysis has a huge impact on the development of chiral new drugs, which has been used in the fields of pharmacy, pesticides, chemicals, materials, and so on. The 2021 Nobel Prize in Chemistry was awarded to German chemist Benjamin List and American chemist David W. C. MacMillan for their pioneering and important contributions to this field. This article will briefly describe chirality and asymmetric catalysis, especially, the history of organocatalysis development, its advantages and future prospects.Key Words: Chirality; Asymmetric catalysis; Organic small molecule catalysis; Nobel prize in chemistry1 2021年诺贝尔化学奖获得者简介2021年10月6日,长期被戏称为“理综奖”的诺贝尔化学奖被授予“对于有机小分子不对称催化[1]的重要贡献”的两位化学家,分别是德国化学家本杰明∙利斯特(Benjamin List)和美国化学家戴维∙麦克米伦(David W. C. MacMillan)。
The Chemistry of Green Synthesis and Catalysis
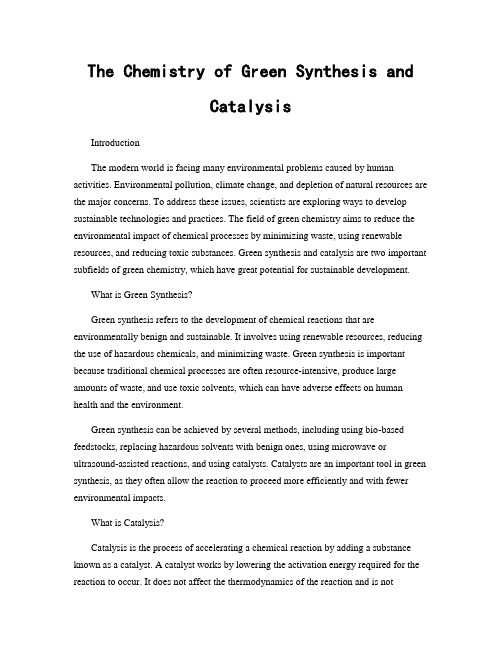
The Chemistry of Green Synthesis andCatalysisIntroductionThe modern world is facing many environmental problems caused by human activities. Environmental pollution, climate change, and depletion of natural resources are the major concerns. To address these issues, scientists are exploring ways to develop sustainable technologies and practices. The field of green chemistry aims to reduce the environmental impact of chemical processes by minimizing waste, using renewable resources, and reducing toxic substances. Green synthesis and catalysis are two important subfields of green chemistry, which have great potential for sustainable development.What is Green Synthesis?Green synthesis refers to the development of chemical reactions that are environmentally benign and sustainable. It involves using renewable resources, reducing the use of hazardous chemicals, and minimizing waste. Green synthesis is important because traditional chemical processes are often resource-intensive, produce large amounts of waste, and use toxic solvents, which can have adverse effects on human health and the environment.Green synthesis can be achieved by several methods, including using bio-based feedstocks, replacing hazardous solvents with benign ones, using microwave or ultrasound-assisted reactions, and using catalysts. Catalysts are an important tool in green synthesis, as they often allow the reaction to proceed more efficiently and with fewer environmental impacts.What is Catalysis?Catalysis is the process of accelerating a chemical reaction by adding a substance known as a catalyst. A catalyst works by lowering the activation energy required for the reaction to occur. It does not affect the thermodynamics of the reaction and is notconsumed in the reaction. Therefore, catalysts can be used repeatedly to speed up reactions without being depleted.Catalysis plays a crucial role in many industrial processes, including the production of fuels, polymers, and pharmaceuticals. However, traditional catalytic processes often use high temperatures and pressures, require toxic solvents, and produce hazardous waste. This is where green catalysis comes in.What is Green Catalysis?Green catalysis is a branch of green chemistry that focuses on developing sustainable catalysts and catalytic processes. Its goal is to reduce the environmental impact of catalytic reactions by using renewable resources, minimizing waste and toxicity, and improving efficiency.Green catalysis has many benefits over traditional catalysis. For example, it can reduce energy consumption and therefore lower greenhouse gas emissions. It can also use non-toxic and renewable resources, which can reduce the environmental impact of the reaction. In addition, green catalysis often uses less hazardous solvents, which improves the safety of the reaction for workers and reduces the risk of contaminated waste.Green Synthesis and Catalysis in ActionThere are many examples of green synthesis and catalysis being used in industrial processes. For example, the production of biodiesel is a green synthesis process that uses vegetable oil or animal fat as a renewable feedstock. The reaction is catalyzed by sodium hydroxide or potassium hydroxide, which are both inexpensive and non-toxic catalysts.Another example is the production of pharmaceuticals using enzyme catalysis. Enzymes are biocatalysts that work under mild conditions of temperature and pressure and produce little or no waste. Their use in the production of pharmaceuticals can reduce the environmental impact of the process and improve the safety for workers.ConclusionGreen synthesis and catalysis are two important subfields of green chemistry that have great potential for sustainable development. By using renewable resources, minimizing waste and toxicity, and improving efficiency, these processes can reduce the environmental impact of chemical reactions. Green synthesis and catalysis are already being used in many industrial processes, and their use is likely to increase in the future as the demand for sustainable technologies and practices grows.。
催化作用基础英文版

催化作用基础英文版Catalysis: A Fundamental ConceptCatalysis is a fundamental concept in chemistry and plays a crucial role in various chemical reactions. It involves the use of a catalyst, a substance that increases the rate of a chemical reaction without being consumed in the process. The word "catalysis" originates from the Greek word "katalysis," which means "dissolution" or "loosening."The concept of catalysis was first introduced by the Swedish chemistJöns Jacob Berzelius in the early 19th century. He observed that certain substances could accelerate chemical reactions without undergoing any permanent changes themselves. This discovery laid the foundation for the study of catalysis and its applications in various fields.Catalysts work by providing an alternative reaction pathway with lower activation energy. Activation energy is the energy required for a chemical reaction to occur. By lowering the activation energy, catalysts enable reactions to proceed at a faster rate. They achieve this by stabilizing the transition state of the reaction, which is an intermediate state between the reactants and the products.There are two types of catalysts: homogeneous catalysts and heterogeneous catalysts. Homogeneous catalysts are in the same phase as the reactants, while heterogeneous catalysts are in a different phase. Homogeneous catalysts are often used in solution-phase reactions, while heterogeneous catalysts are commonly employed in gas-phase or solid-phase reactions.Catalysis finds applications in various industries, including petroleum refining, pharmaceuticals, and environmental protection. In petroleum refining, catalysts are used to convert crude oil into useful products such as gasoline, diesel, and jet fuel. The use of catalysts in this process increases the efficiency of the refining process and reduces the environmental impact.In the pharmaceutical industry, catalysts are employed in the synthesis of drugs. They enable the production of complex molecules with high selectivity and efficiency. Catalysts also play a crucial role in environmental protection. For example, catalytic converters in automobiles convert harmful pollutants into less harmful substances, reducing air pollution.Catalysis is not limited to industrial applications; it also occurs in biological systems. Enzymes, which are biological catalysts, are essential for various biochemical reactions in living organisms. They enable reactions to occur under mild conditions, such as body temperature, which would otherwise require harsh conditions.The study of catalysis has led to significant advancements in the field of chemistry. Researchers continue to explore new catalysts and develop more efficient catalytic processes. The development of sustainable and environmentally friendly catalysts is also a focus of current research.In conclusion, catalysis is a fundamental concept in chemistry that involves the use of catalysts to increase the rate of chemical reactions. It has applications in various industries and plays a crucial role in environmental protection. The study of catalysis has led to significant advancements in chemistry and continues to be an active area of research.。
生物催化剂
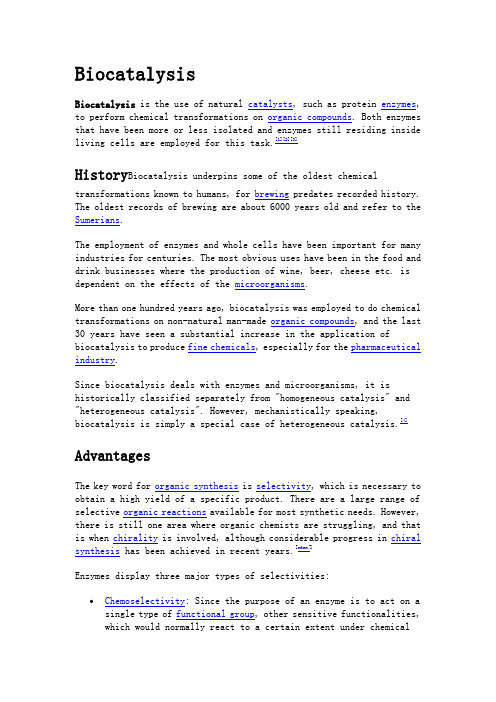
BiocatalysisBiocatalysis is the use of natural catalysts, such as protein enzymes, to perform chemical transformations on organic compounds. Both enzymes that have been more or less isolated and enzymes still residing inside living cells are employed for this task.[1][2][3]History Biocatalysis underpins some of the oldest chemicaltransformations known to humans, for brewing predates recorded history. The oldest records of brewing are about 6000 years old and refer to the Sumerians.The employment of enzymes and whole cells have been important for many industries for centuries. The most obvious uses have been in the food and drink businesses where the production of wine, beer, cheese etc. is dependent on the effects of the microorganisms.More than one hundred years ago, biocatalysis was employed to do chemical transformations on non-natural man-made organic compounds, and the last 30 years have seen a substantial increase in the application of biocatalysis to produce fine chemicals, especially for the pharmaceutical industry.Since biocatalysis deals with enzymes and microorganisms, it is historically classified separately from "homogeneous catalysis" and "heterogeneous catalysis". However, mechanistically speaking, biocatalysis is simply a special case of heterogeneous catalysis.[4]AdvantagesThe key word for organic synthesis is selectivity, which is necessary to obtain a high yield of a specific product. There are a large range of selective organic reactions available for most synthetic needs. However, there is still one area where organic chemists are struggling, and that is when chirality is involved, although considerable progress in chiral synthesis has been achieved in recent years.[when?]Enzymes display three major types of selectivities:Chemoselectivity: Since the purpose of an enzyme is to act on a single type of functional group, other sensitive functionalities, which would normally react to a certain extent under chemicalcatalysis, survive. As a result, biocatalytic reactions tend to be "cleaner" and laborious purification of product(s) from impurities emerging through side-reactions can largely be omitted.∙Regioselectivity and diastereoselectivity: Due to their complex three-dimensional structure, enzymes may distinguish betweenfunctional groups which are chemically situated in differentregions of the substrate molecule.∙Enantioselectivity: Since almost all enzymes are made from L-amino acids, enzymes are chiral catalysts. As a consequence, any type of chirality present in the substrate molecule is "recognized" upon the formation of the enzyme-substrate complex. Thus a prochiralsubstrate may be transformed into an optically active product and both enantiomers of a racemic substrate may react at differentrates.These reasons, and especially the latter, are the major reasons why synthetic chemists have become interested in biocatalysis. This interest in turn is mainly due to the need to synthesise enantiopure compounds as chiral building blocks for drugs and agrochemicals.Another important advantage of biocatalysts are that they are environmentally acceptable, being completely degraded in the environment. Furthermore the enzymes act under mild conditions, which minimizes problems of undesired side-reactions such as decomposition, isomerization, racemization and rearrangement, which often plague traditional methodology.Asymmetric biocatalysisThe use of biocatalysis to obtain enantiopure compounds can be divided into two different methods:1.Kinetic resolution of a racemic mixture2.Biocatalysed asymmetric synthesisIn kinetic resolution of a racemic mixture, the presence of a chiral object (the enzyme) converts one of the enantiomers into product at a greater reaction rate than the other enantiomer.The racemic mixture has now been transformed into a mixture of two different compounds, making them separable by normal methodology. The maximum yield in such kinetic resolutions is 50%, since a yield of more than 50% means that some of wrong isomer also has reacted, giving a lower enantiomeric excess. Such reactions must therefore be terminated before equilibrium is reached. If it is possible to perform such resolutions under conditions where the two substrate- enantiomers are racemizing continuously, all substrate may in theory be converted into enantiopure product. This is called dynamic resolution.In biocatalysed asymmetric synthesis, a non-chiral unit becomes chiral in such a way that the different possible stereoisomers are formed in different quantities. The chirality is introduced into the substrate by influence of enzyme, which is chiral. Yeast is a biocatalyst for the enantioselective reduction of ketones.The biocatalytic Baeyer-Villiger oxidation is another example of a biocatalytic reaction. In one study a specially designed mutant of Candida Antarctica was found to be an effective catalyst for the Michael addition of acrolein with acetylacetone at 20°C in absence of additional solvent.[5]Another study demonstrates how racemic nicotine (mixture of S andR-enantiomers 1 in scheme 3) can be deracemized in a one-pot procedure involving a monoamine oxidase isolated from Aspergillus niger which is able to oxidize only the amine S-enantiomer to the imine2and involving an ammonia–borane reducing couple which can reduce the imine 2 back to the amine 1.[6]In this way the S-enantiomer will continuously be consumed by the enzyme while the R-enantiomer accumulates. It is even possible to stereoinvert pure S to pure R.Biocatalysis application prospectMedicinal compounds are generally and the body of enzymes and proteins or other functional biological macromolecules interaction of activity occurred specific small molecules. Therefore, in the manufacturing process of drug molecules are introducing enzyme as a catalyst is easy to understand. However, to take the nature widespread biological catalytic process into efficient industrial production process, whether depends not only on technology and found that target molecules (most for synthetic unnatural compounds) effective combination of enzyme catalysis and develop, but also on economic, relative to other biological catalysis and process the process route (such as chemical synthesis or microorganism fermentation) competitive advantage. Therefore, relatively small production scale and purity to demand higher drug production naturally become biocatalysis the first target of industrialized application.。
催化剂设计开发
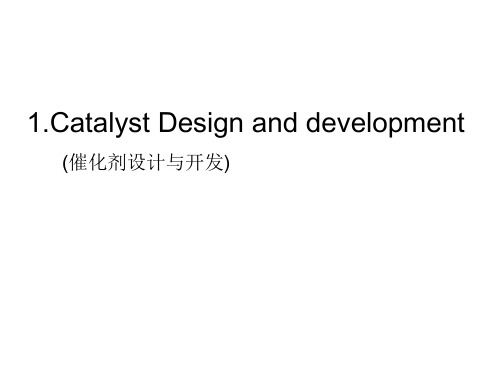
Definitions of Catalysis / catalyst /Turnover
• A catalyst is a material that converts reactants into products, through a series of elementary steps, in which the catalyst participates while being regenerated to its original form at the end of each cycle during its lifetime.
Steps in a Heterogeneous Catalytic Reaction
nanometer length scale.
• surface compohat of the bulk.
• electronic atomic defects. impurities.
• surface atomic structure and composition may change with time-on-stream as the catalytic reaction proceed.
• easy to separate. • the active sites (or active centers) at the
surface of the solid. • The catalyst is typically a high-surface are
a material (e.g., 10–1000 m2g-1). • different types of surface sites. • most catalytic solids are polycrystalline. • most contain particles with sizes in the
15-Homogeneous Catalysis
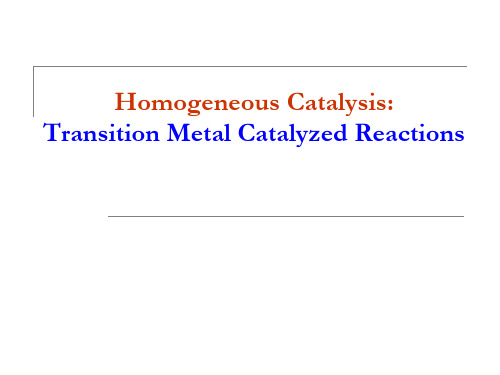
H + H H
OH + R' H H R C R'
H
O R C
H
Many transition metal complexes catalyze such reactions. e.g.
Ph3P Ph3P
Rh
PPh3 Cl
Wilkinson's catalyst
+ Rh Ph3P S = solv ent
A. Introduction to homogeneous catalysis
Catalysts containing d-block metals are of immense importance to the chemical industry. The search for new catalysts is one of the major driving forces behind organometallic research. Catalysts fall into two categories. A homogeneous catalyst is in the same phase as the reactants (normally in solution); a heterogeneous catalyst is in a different phase (usually solid) from the reactants. Heterogeneous catalysts are difficult to characterize. Catalytic mechanisms are considerably easier to study in solution where such powerful methods as NMR can be used to both assign structures and follow reaction kinetics. In this part, we are going to look at some homogeneously catalyzed organic reactions.
Introduction to Natural Products Chemistry
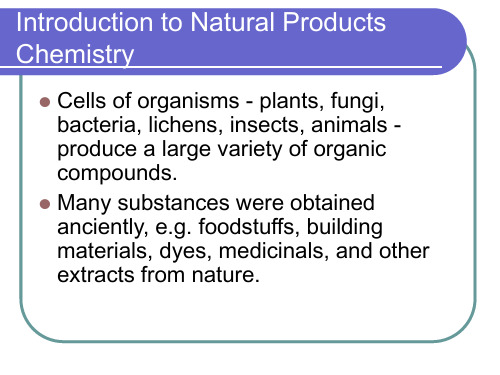
Introduction to Natural Products Chemistry
Plants are particularly interesting because: They have the broadest spectrum of
biosynthetic capability, and produce a wide variety of compounds. They use simple starting materials: water, carbon dioxide, nitrogen (elemental and in salts), phosphorus compounds, and salts. Their biosynthetic paths are known
Introduction to Natural Products Chemistry
In the late 1700's, chemists moved from myth and mystery to basics of modern scientific methods to begin to uncover the true properties of natural extracts from biological systems.
In the 1800's, organic chemistry was exclusively the study of natural products.
Introduction to Natural Products Chemistry
Natural extracts were subjected to separation into component compounds, which were then purified and analyzed.
异构催化剂生产生物柴油1111
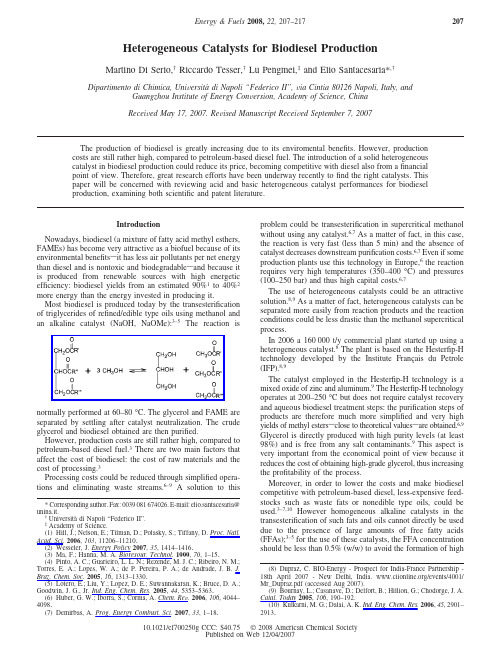
Heterogeneous Catalysts for Biodiesel ProductionMartino Di Serio,†Riccardo Tesser,†Lu Pengmei,‡and Elio Santacesaria*,†Dipartimento di Chimica,Uni V ersitàdi Napoli “Federico II”,V ia Cintia 80126Napoli,Italy,andGuangzhou Institute of Energy Con V ersion,Academy of Science,ChinaRecei V ed May 17,2007.Re V ised Manuscript Recei V ed September 7,2007The production of biodiesel is greatly increasing due to its enviromental benefits.However,production costs are still rather high,compared to petroleum-based diesel fuel.The introduction of a solid heterogeneous catalyst in biodiesel production could reduce its price,becoming competitive with diesel also from a financial point of view.Therefore,great research efforts have been underway recently to find the right catalysts.This paper will be concerned with reviewing acid and basic heterogeneous catalyst performances for biodiesel production,examining both scientific and patent literature.IntroductionNowadays,biodiesel (a mixture of fatty acid methyl esthers,FAMEs)has become very attractive as a biofuel because of its environmental benefits s it has less air pollutants per net energy than diesel and is nontoxic and biodegradable s and because it is produced from renewable sources with high energetic efficiency:biodiesel yields from an estimated 90%1to 40%2more energy than the energy invested in producing it.Most biodiesel is produced today by the transesterification of triglycerides of refined/edible type oils using methanol and an alkaline catalyst (NaOH,NaOMe):3–5The reaction isnormally performed at 60–80°C.The glycerol and FAME are separated by settling after catalyst neutralization.The crude glycerol and biodiesel obtained are then purified.However,production costs are still rather high,compared to petroleum-based diesel fuel.3There are two main factors that affect the cost of biodiesel:the cost of raw materials and the cost of processing.3Processing costs could be reduced through simplified opera-tions and eliminating waste streams.6–9A solution to thisproblem could be transesterification in supercritical methanol without using any catalyst.6,7As a matter of fact,in this case,the reaction is very fast (less than 5min)and the absence of catalyst decreases downstream purification costs.6,7Even if some production plants use this technology in Europe,6the reaction requires very high temperatures (350–400°C)and pressures (100–250bar)and thus high capital costs.6,7The use of heterogeneous catalysts could be an attractive solution.8,9As a matter of fact,heterogeneous catalysts can be separated more easily from reaction products and the reaction conditions could be less drastic than the methanol supercritical process.In 2006a 160000t/y commercial plant started up using a heterogeneous catalyst.8The plant is based on the Hesterfip-H technology developed by the Institute Français du Petrole (IFP).8,9The catalyst employed in the Hesterfip-H technology is a mixed oxide of zinc and aluminum.9The Hesterfip-H technology operates at 200–250°C but does not require catalyst recovery and aqueous biodiesel treatment steps:the purification steps of products are therefore much more simplified and very high yields of methyl esters s close to theoretical values s are obtained.6,9Glycerol is directly produced with high purity levels (at least 98%)and is free from any salt contaminants.9This aspect is very important from the economical point of view because it reduces the cost of obtaining high-grade glycerol,thus increasing the profitability of the process.Moreover,in order to lower the costs and make biodiesel competitive with petroleum-based diesel,less-expensive feed-stocks such as waste fats or nonedible type oils,could be used.3–7,10However homogeneous alkaline catalysts in the transesterification of such fats and oils cannot directly be used due to the presence of large amounts of free fatty acids (FFAs);3–5for the use of these catalysts,the FFA concentration should be less than 0.5%(w/w)to avoid the formation of high*Corresponding author.Fax:0039081674026.E-mail:elio.santacesaria@unina.it.†Universitàdi Napoli “Federico II”.‡Academy of Science.(1)Hill,J.;Nelson,E.;Tilman,D.;Polasky,S.;Tiffany,D.Proc.Natl.Acad.Sci.2006,103,11206–11210.(2)Wesseler,J.Energy Policy 2007,35,1414–1416.(3)Ma,F.;Hanna,M.A.Bioresour.Technol.1999,70,1–15.(4)Pinto,A.C.;Guarieiro,L.L.N.;Rezende,M.J.C.;Ribeiro,N.M.;Torres,E.A.;Lopes,W.A.;de P.Pereira,P.A.;de Andrade,J.B.J.Braz.Chem.Soc.2005,16,1313–1330.(5)Lotero,E.;Liu,Y.;Lopez,D.E.;Suwannakaran,K.;Bruce,D.A.;Goodwin,J.G.,Jr.Ind.Eng.Chem.Res.2005,44,5353–5363.(6)Huber,G.W.;Iborra,S.;Corma,A.Chem.Re V .2006,106,4044–4098.(7)Demirbas,A.Prog.Energy Combust.Sci.2007,33,1–18.(8)Dupraz,C.BIO-Energy -Prospect for India-France Partnership -18th April 2007-New Delhi,/events/4001/Mr_Dupraz.pdf (accessed Aug 2007).(9)Bournay,L.;Casanave,D.;Delfort,B.;Hillion,G.;Chodorge,J.A.Catal.Today 2005,106,190–192.(10)Kulkarni,M.G.;Dalai,A.K.Ind.Eng.Chem.Res.2006,45,2901–2913.Energy &Fuels 2008,22,207–21720710.1021/ef700250g CCC:$40.75 2008American Chemical SocietyPublished on Web 12/04/2007soap concentrations as a consequence of the reaction of FFAs with the basic catalyst:R-COOH +NaOH f R-COOHNa +H 2OThe soap causes downstream processing problems in product separation because of emulsion formation.3,6Several methods have been proposed to solve these prob-lems,11but the most useful seem to be the following:5,10,12–15(a)use of enzymes.A Lypase enzyme catalyzes both transesterification of triglycerides and esterification of FFAR-COOH +MeOH a R-COOMe +H 2Oin one step.9,11–13(b)use of acid catalysts.Acid catalyst can also promote esterification and transesterification.5,10,15(c)pre-esterification method.FFAs are first esterified to FAMEs using an acid catalyst,and then,transesterification is performed,as usual,by using an alkaline catalyst.5,10Enzyme-based transesterification is carried out at moderate temperatures with high yields,but this method cannot be used in industry today due to high enzyme costs,and the problems related to its deactivation caused by feed impurities.10,12–14The enzyme can be immobilized on a support to obtain a heterogeous catalyst,but again,the use will only be possible if the enzyme costs are reduced s as in the case of enzyme use in detergents,dairy products,textile,and leather processing.12However,the use of enzymes is not explored in this review which will be devoted to the use of heterogeneous acid and basic catalysts.More information on the use of enzymes in biodiesel production can be found in recent papers.10,12–14Methods b and c seem to be more attractive.Concerning method b s use of an acid catalyst for both esterification and transesterification reactions s Zhang et al.16,17recently showed,by a technological assessment of different continuous processes,that the homogeneous H 2SO 4-catalyzed process using waste oil is technically feasible and less complex than a two-step process (pre-esterification with homogeneous acid catalyst and alkali-catalyzed steps).However,this process gives rise to problems linked with the corrosive action of the liquid acid catalyst and to the high quantity of byproduct obtained.5,15Basu and Norris 18and more recently Di Serio et al.15and Siano et al.19showed the possibility to perform triglyceride (TG)transesterification and FFA esterification in a single step,using low concentrations of Lewis acid homoge-neous catalysts (carboxylic acid of particular metals).However,this process also gives rise to problems linked with the need to remove catalysts from products by downstream purification.15A solid acid catalyst could eliminate these problems.5,8The homogeneous acid-catalyzed pre-esterification of FFA s method c s is a common practice in reducing FFA levels in high FFA feedstock,before performing the base-catalyzed transesteri-fication.4,5,10The main drawback of the pre-esterification method c consists again in the necessity to remove the homogeneous acid catalyst from the oil after pre-esterification.So for improving the process,the solution is again the use of a heterogeneous esterification catalyst.5From the above discussion,it is clear that the introduction of a solid catalyst in biodiesel production could reduce its price,so biodiesel could become competitive with diesel also from an economic point of view.Therefore,great research efforts have been underway recently to find the right catalysts.Several general reviews on biofuels and biodiesel production have been published:3–7,10one concerns both homogeneous and heterogeneous acid catalysts.5In the second part of a general review on biodiesel production,Lotero et al.20also included an in-depth review on heterogeneous catalysts used in transesteri-fication reactions.Together with the scientific and patent literature concerning biodiesel production,in some cases,we have also considered results reported on the transesterification or esterification of model molecules like triacetin or acetic acid to explain the reaction mechanisms and catalytic behavior of heterogeneous catalysts.However,it must be pointed out that in some cases the data obtained with model molecules cannot be used to predict the behavior of oils/fats and fatty acids because the polar and steric effects of the alpha-substituent group can greatly influence the reactivity.21Heterogenous CatalystsReaction Mechanisms:A General Overview.Heteroge-neous acid and basic catalysts could be classified as Brönsted or Lewis catalysts,though in many cases both types of sites could be present and it is not easy to evaluate the relative importance of the two types of sites in the reaction.A detailed description of some reaction mechanisms can be found in the paper of Lotero et al.20Here for brevity’s sake,we will report only a general overview on reaction mechanisms of the different catalyst types.When homogeneous Brönsted basic catalysts,i.e.,NaOH,KOH,Na 2CO 3,were mixed with alcohol,the actual catalyst is formed.This is the alkoxide group:Na +OH -+CH 3OH f H 2O +CH 3O -Na +which attacks the carbonyl carbon atom of the triglyceride molecule.3,20Often an alkoxide (NaOCH 3,KOCH 3)is directly used as catalyst.A similar mechanism is operative in the case of a heteroge-neous basic Bronsted catalyst such as basic zeolite:20Also inthis case,the formed catalytic specie is a homogeneous alkoxide.In the case of heterogeneous basic Bronsted catalyst such as resin with quaternary ammonium functionality (QN +OH -),the positive counterions (organic ammonium groups),being bonded(11)Ono,T.,Yoshiharu,K.Process for producing lower alcohol esters or fatty acids.U.S.Patent 4,164,506,Aug 14,1979.(12)Fukuda,H.;Kondo,A.;Noda,H.J.Biosci.Bioeng.2001,92,405–416.(13)Haas,M.J.;Piazza,G.J.;Foglia,T.A.Lipid Biotechol.2002,587–598.(14)Kumari,V.;Shah,S.;Gupta,M.N.Energy Fuels 2007,21,368–372.(15)Di Serio,M.;Tesser,R.;Dimiccoli,M.;Cammarota,F.;Nastasi,M.;Santacesaria,E.J.Mol.Catal.A:Chem.2005,239,111–115.(16)Zhang,Y.;Dubè,M.A.;McLean,D.D.;Kates,M.Bioresour.Technol.2003,89,1–16.(17)Zhang,Y.;Dubè,M.A.;McLean,D.D.;Kates,M.Bioresour.Technol.2003,90,229–240.(18)Basu,H.N.;Norris,M.E.U.S.Patent 5,525,126,June 11,1996.(19)Siano,D.;Di Serio,M.;Tesser,R.;Dimmicoli,M.;Cammarota,F.;Santacesaria,E.;Siano,L.;Nastasi,M.Process for the production of esters from V egetables oils or animal fats.PCT No.WO2006/006033,Jan 19,2006.(20)Lotero,E.;Goodwin,J.G.,Jr.;Bruce,D.A.;Suwannakarn,K.;Liu,Y.;Lopez,D.E.Catalysis 2006,19,41–84.(21)Liu,Y.;Lotero,E.;Goodwin,J.G.,Jr.J.Catal.2006,243,221–228.208Energy &Fuels,Vol.22,No.1,2008Di Serio et al.directly to the support surface,electronically retain the catalytic anions on the solid surface:The reaction occurs betweenmethanol adsorbed on the cation and ester from the liquid (Eley–Rideal mechanism).The formation of alkoxide groups is also a fundamental step for heterogeneous basic Lewis catalyst.For example,in the case of ethylacetate transesterification,catalyzed by MgO,the reaction occurs between the methanol molecules adsorbed on a magnesium oxide free basic sites and the ethyl acetate moleculesfrom the liquid phase (Eley–Rideal mechanism).22,23Both homogeneous Brönsted (H 2SO 4,p -toluensolfonic acid 5,20)and Lewis (metal acetate,10,15,18–20metal complexes 24)acid catalysts have been used in biodiesel synthesis,and both catalyze either transesterification and esterification reactions.25Brönsted acid catalysts are active mainly in esterification reactions while Lewis acid catalysts are more active in transesterification reactions (see,for example,Table 126).Table 1reports data of runs performed by using a series of small stainless steel vial reactors.Both the reagents (oil (FFA )0.2%w/w))2.0g,methanol )0.88g)and a specified amount of the catalyst were introduced into each reactor.All the reactors were then heated in a ventilated oven.The temperature of the oven was initially fixed at 50°C for 14min and then increased at a rate of 20°C/min until the reaction temperature was reached,where the samples were kept for the fixed reaction time.At the end of the reaction,the temperature was quenched by putting the vials in a cold bath.Experimental runs were also performed by adding oleic acid to the reaction mixture.Oleic acid has been chosen as a test molecule for simulating the behavior of FFA.The lead acetate (Lewis acid)has greater transesterification activity than p -toluenesulfonic (Brønsted acid)acid,while on the contrary p -toluenesulfonic acid is more active than lead acetate in esterification reactions.The lower yield obtained with lead acetate using an acid oil is justified by the strong deactivation of the Lewis catalyst due to the water formed in the esterification reaction.15In both homogeneous and heterogeneous Brønsted acid catalysis,the mechanism pathways proceed through the protonation of the carbonyl group:increasing its electrophi-licity and rendering it more susceptible to alcohol nucleo-philic attack.21In the case of Nafion supported on silica (Nafion SAC-13),Lopez et al.27proposed a mechanistic pathway for triacetin transesterification s a mechanism similar to that accepted for a homogeneous Brønsted acid catalyst.They found activation energy and reaction orders similar to the ones showed by sulfuric acid.27The reaction mechanism in esterification reactions promoted by solid acid Brønsted catalysts is also similar to the homoge-neous one.28Liu et al.,28found that Nafion supported on silica has comparable turnover frequencies (TOF)to H 2SO 4and a similar reaction mechanism in esterification of the liquid acetic acid with methanol.The reaction occurs via a single-site Eley–Rideal mechanism involving a nucleophilic attack between adsorbed carboxylic acid and unadsorbed alchohol as the rate-determining step.28The formation of a more electrophilic species also occurs with homogeneous and heterogeneous Lewis acid catalysts as the first step in the reaction mechanism:15,29–31In this case,therate-determining step depends on the Lewis catalyst’s acid strength.After the Lewis complex formation (stage 1),the alcohol nucleophilic bonding (stage 2),and the new ester formation (stage 3),the new ester desorbs from the Lewis site (stage 4)and the cycle is repeated.If the strength of acidic sites is too high,the desorption of the product is not favored,(22)Dossin,T.F.;Reyniers,M.F.;Marin,G.B.Appl.Cat.B.2006,61,35–45.(23)Dossin,T.F.;Reyniers,M.F.;Berger,R.J.;Marin,G.B.Appl.Cat.B.En V iron.2006,67,136–148.(24)Abreu,F.R.;Lima,D.G.;Hamù,D.G.;Einloft,S.;Rubim,J.C.;Suarez,P.A.Z.J.Am.Oil Chem.Soc.2003,80,601–604.(25)López,D.E.;Suwannakarn,K.;Bruce,D.A.;Goodwin,J.G.,Jr.J.Cat.2007,247,43–50.(26)DiSerio,M.;Tesser,R.;Mandato,N.;Santacesaria,E.Unpublished data.(27)López,D.E.;Goodwin,J.G.,Jr.;Bruce,D.A.J.Cat.2007,245,381–391.(28)Liu,Y.;Lotero,E.;Goodwin,J.G.,Jr.J.Cat.2006,242,278–286.(29)Parshall,G.W.Ittel SI Homogeneous Catalysis:The Applications and Chemistry of Catalysis by Soluble Transition Metal ,2nd ed.;Wiley-Interscience:New York,2005.(30)Di Serio,M.;Apicella,B.;Grieco,G.;Iengo,P.;Fiocca,L.;Po,R.;Santacesaria,E.J.Mol.Cat.A.Chem.1998,130,233–240.(31)Bonelli,B.;Cozzolino,M.;Tesser,R.;Di Serio,M.;Piumetti,M.;Garrone,E.;Santacesaria,E.J.Cat.2007,246,293–300.Table 1.Oil )Soybean,8g;Methanol/Oil Molar Ratio )12:126catalyst initial FFA conc (%wt)reaction temp (°C)reaction time (min)cat (mol)FAME yield (%)final FFA conc (%wt)uncatalyzed 180402uncatalyzed 20.5180602210.7Pb(Ac)218040 5.4710-592p -toluenesulfonic acid 18040 5.3510-529Pb(Ac)220.518060 5.3810-557 6.8p -toluenesulfonic acid20.5180606.4010-5481.1Catalysts for Biodiesel Production Energy &Fuels,Vol.22,No.1,2008209determining a slow reaction rate.15,29–31This mechanism was confirmed for both homogeneous15,29,30and heterogeneous catalysts31by the observation that an optimal range of strength for Lewis acidic sites exists and that very strong Lewis acidic catalysts are less active in transesterification reactions.15,29–31 Basic Catalysts.Gryglewicz32investigated the possibility of using alkaline-earth metal hydroxides,oxides,and alkoxides to catalyze the transesterifiction of rapeseed oil at methanol reflux temperature.He found that sodium hydroxide was the most active,barium hydroxide was slightly less active,and that calcium methoxide showed medium activity.The reaction rate was lowest when CaO powder was used as catalyst while magnesium oxide and calcium hydroxide showed no catalytic activity.32The high activity of barium hydroxide is due to its higher solubility in methanol with respect to other compounds. The order of reactivity Ca(OH)2<CaO<Ca(CH3O)2agrees with the Lewis basic theory:the methoxides of alkaline-earth metals are more basic than their oxides which are more basic than their hydroxides.32Table2reports some data about CaO catalytic performance.32,33The yield of biodiesel using CaO as a catalyst increases with increase in the temperature and methanol/oil molar ratio,especially in the case of the methanol supercritical state33,34s see Table3entries1–3.In the methanol supercritical state,good performances were obtained also with Ca(OH)2and CaCO334s see Table3entries4–6.Increases in CaO performances can be obtained using nanocrystalline calcium oxides.35The nanocrystalline calcium oxides(crystal size)20nm;specific surface area)90m2/g) give100%conversion of soybean oil at room temperature after 12h while the conversion obtained with commercial CaO (crystal size)43nm;specific surface area)1m2/g)is only 2%.35López Granados et al.36studied the activity of activated CaO as a catalyst in the production of biodiesel by the transesteri-fication of triglycerides with methanol.The active surface sites of CaO are poisoned by the atmospheric H2O and CO2.The catalytic activity of CaO can be improved if CaO is subjected to an activation treatment at high temperature(g700°C)before the reaction s to remove the main poisoning species(the carbonate groups)from the surface s and if the contact with atmospheric air is prevented after this treatment.36Even if the catalyst can be reused for several runs without significant deactivation,dissolution of CaO does occur.The catalytic reaction is the result of the contribution of both heterogeneous and homogeneous catalysis for the formation of leached active species and further investigation is necessary to quantify this aspect.36The transesterification rate can be increased using microwave energy,37,38because the microwave energy selectively energizes the catalyst’s interaction with the reactants.38Table4(entries1and2)reports results obtained with Ca(OH)238and Ba(OH)2.37The data of Mazzochia et al.37 confirm that Ba(OH)2is not a completely heterogeneous catalyst. As a matter of fact,when the product obtained after reaction is not washed several times with distilled water,the resulting FAME and glycerine contain ca.0.06%and0.25%of barium, respectively.Good results in transesterification of soybean oil were obtained using ZnO loaded Sr(NO3)2followed by calcination at873K for5h.39When the transesterification reaction was carried out at reflux of methanol(65°C),with a12:1molar ratio of methanol to soybean oil and a catalyst amount of5wt %,the conversion of soybean oil was94.7%.39The SrO derived from thermal decomposition of Sr(NO3)2at high calcination temperatures is probably the main catalytically active specie.39 However,the used catalyst was significantly deactivated and could not be directly reused for transesterification.39Yang and Xie39explained the deactivation by the deposition of reactants and products on the active sites and/or by a transformation of the active sites and their interactions during the reaction. However,the leaching of SrO was not examined;notwithstand-ing,its high solubility in the reaction environment36is known. Sodium silicate catalyzes the transesterification of oils with high rates at moderate temperatures(60–120°C)s even if no data on catalyst reusability was reported.38Also in this case, the use of microwave energy and high methanol/oil ratio greatly increases the performances s see Table4entries3and4. Corma et al.,40in a patent mainly devoted to the transesteri-fication of triglycerides with glycerol to prepare monoglycerides, claimed the possibility to use calcined hydrotalcites and magnesium oxides in promoting the transesterification of triglycerides with monoalcohols,even if no examples or experimental data for this reaction are reported in the mentioned patent.Leclercq et al.41tested the use of commercial MgO/Al2O3 hydrotalcites and MgO(300m2/g)in the transesterification of rapeseed oil.They found that MgO was more active than hydrotalcite s see Tables5and6entry1.On the other hand, Cantrell et al.,42successfully used calcined hydrotalcites in promoting the transesterification of glycerol tributyrate with methanol at60°C.The rate increases steadily with Mg content, and the most active catalyst Al/(Mg+Al))0.25was10times more active than MgO.42Xie et al.,43for soybean oil transes-terification at methanol reflux,found that the most active calcined hydrotalcite has again an atomic ratio Al/(Mg+Al) )0.25s see Table6entries2–4s and that a higher active solid is obtained by calcining it at500°C;see Table6entries3,5, and6.Di Serio et al.44and Siano et al.45showed the possibility(32)Gryglewicz,S.Bioresour.Technol.1999,70,249–253.(33)Demirbas,A.Energy Con V ers.Manage.2007,48,937–941.(34)Tateno T.,Sasaki T.Process for producing fatty acid fuels comprising fatty acids esters.U.S.Patent6,818,026,Nov16,2004.(35)Reddy,C.;Reddy,V.;Oshel,R.;Verkade,J.G.Energy Fuels2006, 20,1310–1314.(36)Lopez Granados,M.;Zafra Poves,M.D.;Martin Alonso,D.; Mariscal,R.;Cabello Galisteo,F.;Moreno Tost,R.;Santamaria,J.;Fierro, J.L.G.App.Cat.B.2007,73,317–326.(37)Mazzocchia,C.;Modica,G.;Nannicini,R.;Kaddouri,A.C.R. Chim.2004,7,601–605.(38)Portnoff,M.A.;Purta,D.A.;Nasta,M.A.;Zhang,J.Pourarian,F.Methods for producing biodiesel.PCT No.WO2006/002087,Jan5,2006.(39)Yang,Z.Q.;Xie,W.L.Fuel Process.Technol.2007,88,631–638.(40)Corma,A.,Iborra,S.,Miquel,S.,Primo Millo,J.Process and Catalysts for the Selective production of Esters of fatty Acids.PCT No WO98/56747,Dec17,1998.(41)Leclercq,E.;Finiels,A.;Moreau,C.J.Amer.Oil Chem.Soc.2006, 78,1161–1165.(42)Cantrell,D.G.;Gillie,L.J.;Lee,A.F.;Wilson,K.Appl.Catal., A2005,287,183–1990.(43)Xie,W.;Peng,H.;Chen,L.J.Mol.Cat.A.2006,246,24–32.(44)Di Serio,M.;Ledda,M.;Cozzolino,M.;Minutillo,G.;Tesser,R.; Santacesaria,E.Ind.Eng.Chem.Res.2006,45,3009–3014.Table2.FAME Yield Using CaO as Catalyst at15min ofReaction Timeref oilreactiontemp(°C)methanol/oilmolar ratiocat conc(%w/w)FAMEyield(%)32rapeseed methanol reflux 4.5:10.81033sunflower19241.1:1 3.050210Energy&Fuels,Vol.22,No.1,2008Di Serio et al.to use calcined hydrotalcites and MgO for industrial biodiesel production at moderately high temperature.High yields of methyl esters were obtained in1h of reaction time at180–200°C s see Tables5(entries2–3)and6(entry7).At least four different types of basic sites have been indentified on the surface of MgO and calcined hydrotalcite catalysts.44The strongest basic sites(superbasic)promote the transesterification reaction also at very low temperatures(100°C),while the basic sites of medium strength require higher temperatures to promote the same reaction.44The experimental data reported show a correlation not only with the catalyst basicity but also with its structural texture.44However,the structural texture of the catalysts examined is dependent on both the precursor and the preparation method.44,46MgO catalyst used to promote transesterification strongly increases the reaction rate in supercritical conditions,34as can be seen in Table3entry7.As vegetable oils,animal fats,and alcohols usually contain water,44the influence of the presence of water on MgO and calcined hydrotacite performances have also been investigated.44,45 Some runs have been performed at180°C in the presence of high water concentration(10000ppm).44The results show that the activity of both magnesium oxide and calcined hydrotalcite is not affected by the presence of an excess of water.44This lastfinding is relevant for industrial purposes,because the possibility to operate in the presence moisture reduces the raw material pretreatment costs and opens the possibility to use unrefined bioethanol.However,Oku et al.47showed that in a run performed at150°C with60g of triolein,20g of methanol,and2.5g of Mg/Al hydrotalcite after24h of reaction,a FAME yield of77was produced,but a very high concentration of Mg and Al ions were detected in the products(Mg17800ppm;Al6900ppm).So, the problems of catalyst leaching need more in-depth study to confirm the possibility of using MgO and related hydrotalcites as industrial catalysts.Corma et al.48have reported that calcined Li/Al hydrotalcites are more active in glycerolysis of fatty acid methyl esters than the Mg/Al material(or MgO)due to their higher Lewis basicity. Starting from this observation,Shumaker et al.49studied the transesterification of soybean oil to fatty acid methyl esters using a calcined Li/Al layered double hydroxide catalyst.It was found that,at the reflux temperature of methanol,near-quantitative conversion of the soybean oil was achieved at low catalyst loadings(2–3wt%)and short reaction times(∼2h).49Catalyst recycling runs showed that the catalyst maintained a high level(45)Siano,D.;Nastasi,M.;Santacesaria,E.;Di Serio,M.;Tesser,R.; Minutillo,G.;Ledda,M.;Tenore,T.Process for Producing esters from vegetable oils or animal fats using heterogeneous catalysts.PCT Application No WO2006/050925,May18,2006.(46)Choudhary,V.R.;Pandit,M.Y.Appl.Catal.1991,71,265–274.(47)Oku,T.;Nonoguchi,M.;Moriguchi,T.Method of producing of fatty alkyl esters and/or glycerine and fatty acid alkyl ester-containing composition.PCT Application No WO2005/021697,Mar10,2005.(48)Corma,A.;Hamid,S.B.A.;Iborra,S.;Velty,A.J.Catal.2005, 234,340–347.(49)Shumaker,J.L.;Crofcheck,C.;Tackett,S.A.;Santillan-Jimenez,E.;Crocker,M.Cat.Lett.2007,115,56–61.Table3.FAME Yield Using Basic Catalysts in Methanol Supercritical Conditionsentry ref oil catalystreactiontemp(°C)methanol/oilmolar ratioreacttime(min)cat conc(%w/w)FAMEyield(%)133sunflower CaO252 6.0:115 3.065 233sunflower CaO25241.1:115 3.099 334soybean CaO30039.3:1100.5897 434soybean CaCO325039.3:110 1.1487 534soybean CaCO330039.3:1100.6799 634soybean Ca(OH)230039.4:1100.6898 734soybean MgO30039.6:110 1.2991 parison of the Transesterification Tests of Oil with Methyl Alcohol Carried out under Microwave Irradiationentry ref oil catalystreactiontemp(°C)methanol/oilmolar ratioreactiontime(min)cat conc(%w/w)FAMEyield(%)137rapeseed Ba(OH)2methanol reflux9:1150.597–98 238soybean Ca(OH)21006:120 2.081 338castor oil sodium silicate1206:110 1.570 438castor oil sodium silicate12019:110 1.5100Table5.FAME Yield Using MgO as Catalystentry ref oil surfaceareareactiontemp(°C)methanol/triglyceridesmolar ratioreactiontime(h)cat conc(%w/w)FAMEyield(%)141rapeseed300methanol reflux75:1221064 244soybean3618012:11 5.072 344soybean22918012:11 5.090Table6.FAME Yield Using Calcined MgO/Al2O3as Catalystentry ref oil Al/(Mg+Al)surfaceareacalcinationtemp(°C)reactiontemp(°C)methanol/oilmolarratioreactiontime(h)cat conc(%w/w)FAMEyield(%)141rapeseed0.30160450methanol reflux275:1221034 243soybean0.28500methanol reflux15:197.528 343soybean0.25500methanol reflux15:197.566 443soybean0.22500methanol reflux15:197.550 543soybean0.25450methanol reflux15:197.545 643soybean0.25600methanol reflux15:197.557 744soybean0.2514450018012:11 5.092 Catalysts for Biodiesel Production Energy&Fuels,Vol.22,No.1,2008211。
罗氏(英文版)-TUNEL-细胞凋亡原位检测试剂盒-POD

In Situ Cell Death Detection Kit, POD
y Version 14
Content version: July 2012
Kit for immunohistochemical detection and quantification of apoptosis (programmed cell death) at single cell level, based on labeling of DNA strand breaks (TUNEL technology): Analysis by light microscopy.
Cat. No. 11 684 817 910
Store the kit at Ϫ15 to Ϫ25°C
1 Kit (50 tests)
1. 1.1 1. 1.1 1.2 2. 2.1 2.2 3. 3.1 3.2
Preface Table of contents Preface .............................................................................................................................2 Table of contents ..................................................................................................................................... 2 Kit contents ................................................................................................................................................ 3 Introduction .....................................................................................................................5 Product overview ..................................................................................................................................... 5 Background information ....................................................................................................................... 8 Procedures and required materials ...........................................................................9 Flow chart .................................................................................................................................................10 Preparation of sample material ........................................................................................................10 3.2.1 Adherent cells, cell smears and cytospin preparations ..............................................11 3.2.2 Tissue sections ...........................................................................................................................11 3.2.2.1 Treatment of paraffin-embedded tissue ............................................................11 3.2.2.2 Treatment of cryopreserved tissue ......................................................................12 Labeling protocol ...................................................................................................................................13 3.3.1 Before you begin .......................................................................................................................13 3.3.2 Labeling protocol for adherent cells, cell smears, cytospin preparations and tissues ........................................................................................................14 3.3.3 Labeling protocol for difficult tissue ..................................................................................15 Signal conversion ..................................................................................................................................16 Appendix ....................................................................................................................... 17 Troubleshooting .....................................................................................................................................17 References ...............................................................................................................................................20 Ordering guide .......................................................................................................................................21
作业及检索实1
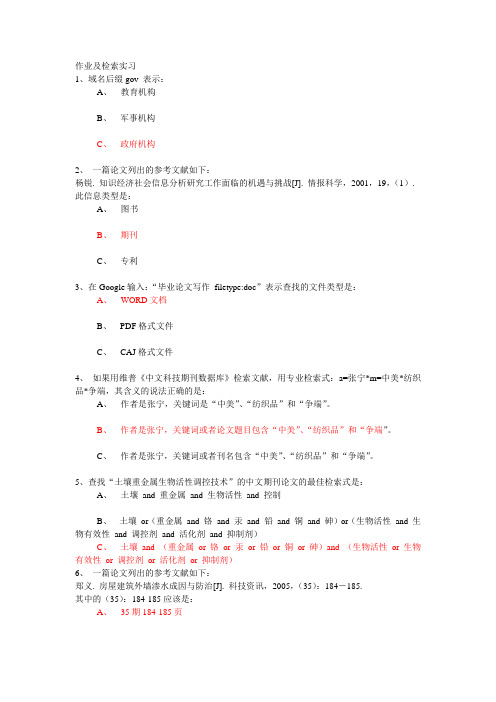
作业及检索实习1、域名后缀gov 表示:A、教育机构B、军事机构C、政府机构2、一篇论文列出的参考文献如下:杨锐. 知识经济社会信息分析研究工作面临的机遇与挑战[J]. 情报科学,2001,19,(1). 此信息类型是:A、图书B、期刊C、专利3、在Google输入:“毕业论文写作filetype:doc”表示查找的文件类型是:A、WORD文档B、PDF格式文件C、CAJ格式文件4、如果用维普《中文科技期刊数据库》检索文献,用专业检索式:a=张宁*m=中美*纺织品*争端,其含义的说法正确的是:A、作者是张宁,关键词是“中美”、“纺织品”和“争端”。
B、作者是张宁,关键词或者论文题目包含“中美”、“纺织品”和“争端”。
C、作者是张宁,关键词或者刊名包含“中美”、“纺织品”和“争端”。
5、查找“土壤重金属生物活性调控技术”的中文期刊论文的最佳检索式是:A、土壤and 重金属and 生物活性and 控制B、土壤or(重金属and 铬and 汞and 铅and 铜and 砷)or(生物活性and 生物有效性and 调控剂and 活化剂and 抑制剂)C、土壤and (重金属or 铬or 汞or 铅or 铜or 砷)and (生物活性or 生物有效性or 调控剂or 活化剂or 抑制剂)6、一篇论文列出的参考文献如下:郑义. 房屋建筑外墙渗水成因与防治[J]. 科技资讯,2005,(35):184-185.其中的(35):184-185应该是:A、35期184-185页B、35卷184-185期C、35期184页185行7、(检出的相关文献/检出的所有文献)×100%的值为:A、查全率B、查准率C、漏检率8、用中国国家知识产权局检索西南大学申请的三峡库区的麻竹(一种苗木)的繁殖方法的专利,选择的检索途径和输入的检索式应该是:A、【名称】西南大学and 三峡库区and 麻竹and 繁殖and 方法B、【名称】三峡库区麻竹繁殖方法【申请(专利权)人】西南大学C、【名称】三峡and 麻竹and 繁殖and 方法【申请(专利权)人】西南大学9、使用分类号检索主要是为了考虑:A、查全率B、查准率C、漏检率10、用维普《中文科技期刊数据库》查询刊物《图书情报知识》的关于数字图书馆的文献,专业检索式为:A、K=数字图书馆+J=图书情报知识B、K=数字图书馆*J=图书情报知识C、K=数字图书馆*A=图书情报知识11、一篇论文的参考文献如下:Chamovitz,Daniel A.;Deng,Xing-Wang.Light Signaling in Plants.Critic Review of Plant Science.1996,15(5-6),455-478.其中数字部分表示:A、1996年15卷5至6期455-478页B、1996年15卷5期455-478页C、1996年15卷5至6期455-478行12、在中国国家知识产权局网站检索康佳公司(简称)申请的带摄像头的手机的专利,例如“一种用带摄像头的手机实现网络摄像头的装置”、“带摄像头手机及其向电脑输入视频数据的方法”、“带旋转摄像头的手机”;要求单词“摄像头”在“手机”的前面,排除名称类似“手机摄像头的旋转调节装置”的相关专利,正确的说法是:在_____字段应输入_____,在_____字段输入:_____A、发明名称,摄像头的手机,地址,康佳公司B、发明名称,摄像头and 手机,地址,康佳公司C、发明名称,摄像头%手机,申请人(专利权人),康佳%公司13、在中国国家知识产权局网站检索西南大学申请的有关生物分子的专利,要求单词“生物”在“分子”的前面,排除名称类似“分子生物”的相关专利,正确的说法是在_____字段应输入_____,在_____字段输入:_____A、发明名称,生物%分子,地址字段,西南大学B、发明名称,生物%分子,申请人/专利权人字段,西南大学C、发明名称,生物+分子,申请人/专利权人字段,西南大学14、使用Google学术搜索检索含有“Cabbage”(甘蓝) ,不包含“Chinese cabbage”(青菜、白菜)的有关文献时,检索式应该这样输入:A、Cabbage not "Chinese cabbage"B、Cabbage -"Chinese cabbage" 备注:减号前面有一个空格。
1.Catalysis (1)
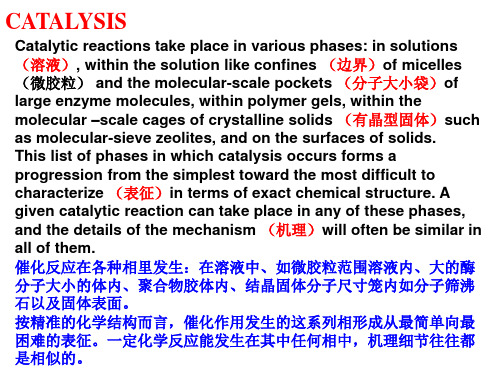
对于一个特定反应,催化剂总是提供一个以上的动力学上有意义反应物的 转换途径。催化剂实际上提供了这种途径作为催化剂与反应物化学键合的 结果。阻抑剂使反应慢下来,一个竞争性的阻抑剂通过与反应物竞争和催 化剂键合。非常强的阻抑剂如此强的键合以至于实际上排除了反应物与催 化剂的键合,其被称作毒物。
CATALYSIS
Catalysis always involves a cycle of reaction steps, and the catalyst is converted from one form to the next, ideally without being consumed in the overall process. The occurrence of a cyclic reaction sequence is a requirement for catalysis, and catalysis may even be defined as such an occurrence.
CATALYSIS
Catalytic reactions take place in various phases: in solutions (溶液), within the solution like confines (边界)of micelles (微胶粒) and the molecular-scale pockets (分子大小袋)of large enzyme molecules, within polymer gels, within the molecular –scale cages of crystalline solids (有晶型固体)such as molecular-sieve zeolites, and on the surfaces of solids.
金属有机Chapter 9

9HOMOGENEOUS CATALYSISThe catalysis of organic reactions1–4is one of the most important applications of organometallic chemistry and has been a significant factor in the rapid devel-opment of the wholefianometallic catalysts have long been used in industrial processes but are now being routinely applied in organic synthetic problems as well.A catalyst is an additive used in substoichiometric amount to bring about a reaction at a temperature below that required for the uncatalyzed thermal reaction.It binds the reactants,or substrates,for the catalytic reaction, causes the desired reaction,and then liberates the reaction products to regenerate the catalyst.The catalyst reenters the catalytic cycle by binding the reagents once more.A typical catalyst may participate in the catalytic cycle101–106times or more,allowing its use in modest or even trace amount.The catalysts we look at are soluble complexes,or homogeneous catalysts,as opposed to catalysts such as palladium on carbon,or heterogeneous catalysts.4These terms are used because the catalyst and substrates for the reaction are in the same phase in the homo-geneous,but not in the heterogeneous,type,where catalysis takes place at the surface of a solid catalyst.Some reactions,such as hydrogenation,are amenable to both types of catalysis,but others are currently limited to one or the other, for example,O2oxidation of ethylene to the epoxide over a heterogeneous silver catalyst or Wacker air oxidation of ethylene to acetaldehyde with homogeneous Pd(II)catalysts.The term homogeneous catalysis also covers simple acid catalysts and non-organometallic catalysis,such as the decomposition of H2O2by Fe2+.Catalytic mechanisms are considerably easier to study in homogeneous systems,where The Organometallic Chemistry of the Transition Metals,Fourth Edition,by Robert H.Crabtree Copyright 2005 John Wiley & Sons, Inc., ISBN 0-471-66256-9235such powerful methods as NMR can be used to both assign structures and follow reaction kinetics.Homogeneous catalysts have the disadvantage that they can be difficult to separate from the product.Sometimes this requires special separation techniques,but in other cases,such as polymer synthesis,the product can be sold with the catalyst still embedded in it.Homogeneous catalysts can also be chemi-cally grafted on to solid supports for greater ease of separation of the catalyst from the reaction products.Although the catalyst is now technically heterogeneous,it often retains the characteristic reactivity pattern that it showed as a homogeneous catalyst,and its properties are usually distinct from those of any of the classical heterogeneous catalysts—these are sometimes called“heterogenized”homoge-neous catalysts.The mechanistic ideas developed in homogeneous catalysis are also becoming more influential in thefield of classical heterogeneous catalysis by suggesting structures for intermediates and mechanisms for reaction steps.By bringing about a reaction at lower temperature,a catalyst can save energy in commercial applications.It often gives higher selectivity for the desired prod-uct,minimizing product separation problems and avoiding the need to discard the undesired product as waste.The selectivity can be changed by altering the ligands,allowing synthesis of products not formed in the uncatalyzed process. With growing regulatory pressure to synthesize drugs in enantiopure form,asym-metric catalysis has come to the fore,along with enzyme catalysis,as the only practical way to make such products on a large scale.Older commercial processes tended to give side products that had to be discarded,such as inorganic salts. Environmental concerns have promoted the idea of atom economy,which values a process most highly when all the atoms in the reagents are used to form the product,minimizing waste.For example,the Monsanto process of Section12.1 is atom economic because it converts MeOH and CO to MeCOOH with no atoms left over.A typical reaction(Eq.9.1)that is catalyzed by many transition metal com-plexes is the isomerization of allylbenzene(the substrate)into propenylbenzene (the product).Normally,the substrate for the reaction will coordinate to the metal complex that serves as catalyst.The metal then brings about the rearrange-ment,and the product dissociates,leaving the metal fragment free to bind a new molecule of substrate and participate in the catalytic cycle once again.Ph(9.1)9.19.2Before setting out tofind a catalyst for a given reaction,say the one shown in Eq.9.1,thefirst consideration is thermodynamic:whether the reaction is favor-able.If the desired reaction were thermodynamically strongly disfavored,as is the conversion of H2O to H2and O2,for example,then no catalyst,however effi-cient,could on its own bring about the reaction.If we wanted to bring about an unfavorable reaction of this sort,we would have to provide the necessary driving force in some way.There are ways of doing this,such as coupling a strongly favorable process to the unfavorable one you want to drive,as Nature commonlydoes with the hydrolysis of ATP(adenosine triphosphate),or we could use the energy of a light photon,as in photosynthesis or we can selectively remove the products(e.g.,by distillation).Normally,the catalyst only increases the rate of a process but does not alter its position of equilibrium,which is decided by the relative thermodynamic stabilities of substrate and products(we discuss ways of getting around this restriction in Section12.4).Fig.9.1a illustrates this point:The substrate S is slightly less stable than the product P,so the reaction will eventually reach an equilibrium favoring P.In the case of9.1going to9.2,the additional conjugation present in9.2is sufficient to ensure that the product is thermodynamically more stable than the starting material and so the reaction is indeed favorable.Normally,the substrate binds to the metal before it undergoes the rearrangement.This substrate–catalyst complex is represented as“M.S”in Fig.9.1.It might be thought that strong binding would be needed.A moment’s reflection will show why this is not so.If the binding is too strong,M.S will be too stable,and the activation energy to get to“M.TS”will be just as large as it was in going from S to TS in the uncatalyzed reaction.S cannot bind too weakly because it may otherwise be excluded from the metal and fail to be activated by the metal at all.Similarly,the product P will normally be formed as the complex M.P.Product P must be the least strongly bound of all because if it is not then S will not be able to displace P,and the catalyst will be effectively poisoned by the products of the reaction.Many of these ideas also apply to the chemistry of Nature’s homogeneous catalysts, enzymes.5Each time the complete catalyst cycle occurs,we consider one catalytic turn-over(one mole of product formed per mole of catalyst)to have been completed. The catalytic rate can be conveniently given in terms of the turnover frequency (TOF)measured in turnovers per unit time(often per hour);the lifetime of the catalyst before deactivation is measured in terms of total turnovers.For most transition metal catalysts,the catalyzed pathway is completely changed from the pathway of the uncatalyzed reaction,as shown in Fig.9.1a.Instead of passing by way of the high-energy uncatalyzed transition state TS,the catalyzed reaction normally goes by a multistep mechanism in which the metal stabilizes intermediates that are stable only when bound to the metal.One new transition state M.TS is shown in Fig.9.1.The TS structure in the absence of the metal would be extremely unstable,but the energy of binding is so high that M.TS is now much more favorable than TS and the reaction all passes through the catalyzed route. Different metal species may be able to stabilize other transition states TS —which may lead to entirely different products—hence different catalysts can give different products from the same starting materials.In a stoichiometric reaction,the passage through M.TS would be the slow, or rate-determining,step.In a catalytic reaction the cyclic nature of the system means that the rates of all steps are identical.On a circular track,on average the same number of trains must pass each point per unit time.The slow step in a catalytic process is called the turnover limiting step.Any change that lowers the barrier for this step will increase the turnover frequency(TOF).Changes inM[M.S][M.P][M.I]irreversible catalyst deactivation product[M ′′]substrate (b )off-loop species[M.S ′]intermediate active catalyst catalyst precursorM ′(a )FIGURE 9.1(a )A catalyst lowers the activation energy for a chemical reaction.Here the uncatalyzed conversion of substrate S to product P passes by way of the high-energy transition state TS.In this case the metal-catalyzed version goes via a different transition state TS ,which is very unstable in the free state but becomes viable on binding to the catalyst as M.TS .The arrow represents the M −TS binding energy.The uncatalyzed and catalyzed processes do not necessarily lead to the same product as is the case here.(b )Typical catalytic cycle in schematic form.other barriers will not affect the TOF.For a high TOF,we require that none of the intermediates be bound too strongly (otherwise they may be too stable and not react further)and that none of the transition states be prohibitively high in energy.Indeed,the whole reaction profile must not stray from a rather narrowALKENE ISOMERIZATION239 range of free energies,accessible at the reaction temperature.Even if all this is arranged,a catalyst may undergo a catalytic cycle only a few times and then“die.”This happens if undesired deactivation reactions are faster than the productive reactions of the catalytic cycle itself.There are many ways in which a catalyst can fail,and for success it is often necessary to look hard for the right metal,ligand set,solvent,temperature range,and other conditions.Many of the reactions that occupied the attention of the early workers were relatively forgiving in terms of the range of possible catalysts and conditions.Some of the problems that are under study today,notably alkane conversions,constitute more searching tests of the efficiency of homogeneous catalysts.Figure9.1b shows a schematic catalytic cycle.The active catalyst M is often rather unstable and is only formed in situ from the catalyst precursor(or precat-alyst),M .If during the reaction we observe the system,for example,by NMR, we normally see only the disappearance of S and the appearance of P.Decreasing the substrate concentration[S]and increasing the metal concentration[M]may allow us to see the complex.We may still see only M because only a small fraction of the metal is likely to be on the loop at any given time.Even if an observed species appears to be an intermediate,we still cannot be sure it is not M·S ,an off-loop species.If a species builds up steadily during the reaction, it might be a catalyst deactivation product M ,in which case the catalytic rate will fall as[M ]rises.Excellent reviews are available on the determination of mechanism in catalytic reactions.39.1ALKENE ISOMERIZATIONMany transition metal complexes are capable of catalyzing the1,3-migration of hydrogen substituents in alkenes,a reaction that has the net effect of moving the C=C group along the chain of the molecule(e.g.,Eq.9.1).This is often a side reaction in other types of catalytic alkene reaction,desired or not according to circumstances.Two mechanisms are most commonly found:thefirst goes via alkyl intermediates(Fig.9.2a);the second,byη3-allyls(Fig.9.2b).Note that in each cycle,all the steps are reversible,so that the substrates and products are in equilibrium,and therefore although a nonthermodynamic ratio of alkenes can be formed at early reaction times,the thermodynamic ratio is eventually formed if the catalyst remains active long enough.In other catalytic reactions,we sometimesfind that the last step is irreversible.As we shall see later,this distinction has important practical consequences in allowing the formation of grossly nonthermodynamic ratios(e.g.,in asymmetric catalysis).Alkyl MechanismIn the alkyl route,we require an M−H bond and a vacant site.The alkene binds and undergoes insertion to give the alkyl.For1-butene,the alkyl might be the1◦or the2◦one,according to the regiochemistry of the insertion.If the1◦alkyl is240HOMOGENEOUS CATALYSIS((FIGURE 9.2The (a )alkyl and (b )allyl mechanisms of alkene isomerization.The open box represents a 2e vacancy or potential vacancy in the form of a labile 2e ligand.formed,βelimination can give back only 1-butene,but βelimination in the 2◦alkyl,often faster,can give both 1-and cis -and trans -2-butene.Since insertion to give the 1◦alkyl is favored for many catalysts,nonproductive cycling of the 1-butene back to 1-butene is common,and productive isomerization may be slower.The initial cis/trans ratio in the 2-butenes formed depends on the catalyst;the cis isomer is often favored.The final ratio depends only on the thermodynamics,and the trans isomer is preferred.A typical isomerization catalyst is RhH(CO)L 3(L =PPh 3).6As this is a coordinatively saturated 18e species it must lose a ligand,PPh 3in this case,to form a coordinatively unsaturated intermediate (<18e),able to bind the alkene.ALKENE HYDROGENATION241 Allyl MechanismThe second common mechanism involves allyl intermediates and is adopted by those metal fragments that have two2e vacant sites but no hydrides.It has been established for the case of Fe3(CO)12as catalyst,a system in which“Fe(CO)3,”formed by fragmentation of the cluster on heating,is believed to be the active species.7The cluster itself is an example of a catalyst precursor.As a14e species, Fe(CO)3may not have an independent existence in solution,but may always be tied up with substrate or product.The open square in Fig.9.2represents a vacant site or a labile ligand.In this mechanism the C−H bond at the acti-vated allylic position of the alkene undergoes an oxidative addition to the metal. The product is anη3-allyl hydride.Now,we only need a reductive elimina-tion to give back the alkene.Again,we can have nonproductive cycling if the H returns to the same site it left,rather than to the opposite end of the allyl group.An experimental distinction7can be made between the two routes with a crossover experiment(Section6.5)using the mixture of C5and C7alkenes of Eq.9.2.For the allyl mechanism,we expect the D in9.3a to end up only in the corresponding product9.3b having undergone an intramolecular1,3shift.For the hydride mechanism,the D will be transferred to the catalyst that can in turn transfer it by crossover to the C5product.DFe(CO)D ++9.3a9.3b(9.2)žCatalytic cycles involve a series of reaction steps of the types seen inChapters5–8.žThe catalyst precursor is usually transformed in some way before enteringthe cycle.žObservable species in the catalytic solution are often off-loop species(Fig9.1).9.2ALKENE HYDROGENATIONHydrogenation catalysts3add molecular hydrogen to the C=C group of an alkene to give an alkane.Three general types have been distinguished,according to242HOMOGENEOUS CATALYSIS the way each type activates H 2.This can happen by (1)oxidative addition,(2)heterolytic activation,and (3)homolytic activation.Oxidative AdditionPerhaps the most important group employs oxidative addition,of which RhCl (PPh 3)3(9.4,Wilkinson’s catalyst)is the best known.A catalytic cycle that is important under certain conditions is shown in Fig.9.3.Hydrogen addition to give a dihydride leads to labilization of one of the PPh 3ligands (high trans effect of H)to give a site at which the alkene binds.The alkene inserts,as in isomerization,but the intermediate alkyl is irre-versibly trapped by reductive elimination with the second hydride to give an alkane.This is an idealized mechanism.3In fact,9.4can also lose PPh 3to give RhCl (PPh 3)2,and dimerize via halide bridges and each of these species have their own separate catalytic cycles 3c that can be important under different condi-tions.Indeed,RhClL 2reacts so much faster with H 2than does RhClL 3that the vast majority of the catalytic reaction goes through RhClL 2under most condi-tions.By reversibility arguments,the more rapid oxidative addition of H 2to the 3-coordinate d 8RhClL 2to give 5-coordinate d 6RhH 2ClL 2relative to the corre-sponding 4-coordinate →6-coordinate conversion is consistent with the tendency for faster reductive elimination from 5-coordinate d 6species that was discussed in Section 6.5.In a key study by Tolman and co-workers 3d ,the dihydride was directly seen by 31P NMR under H 2and the reversible loss of the PPh 3trans to a hydride detected from a broadening of the appropriate resonance,as discussed in Section 10.5.Figure 9.3represents the hydride mechanism in which H 2adds before the olefin.Sometimes the olefin adds first (the olefin mechanism )as is found for [Rh(dpe)(MeOH)2]BF 4.3eRhCl L L H HL Rh ClL L H RhCl L L HLH Rh Cl L H FIGURE 9.3Mechanism for the hydrogenation of alkenes by Wilkinson’s catalyst.Other pathways also operate in this system,however.ALKENE HYDROGENATION243PPh3Cl Rh PPh3PPh39.4Since we need to bind two hydrides and the alkene,for a total electron count of4e,the16e catalyst RhCl(PPh3)3needs to dissociate a ligand,PPh3in this case,to do this.The PEt3analog of9.4reacts with H2to give a stable and cat-alytically inactive dihydride RhH2Cl(PEt3)3,9.5.The smaller PEt3ligand does not dissociate and so9.5is not an active catalyst.All we have to do to make the PEt3analog active is artificially arrange to generate the desired RhH2ClL2 intermediate by forming it in situ by starting with0.5equiv of[(nbd)Rh(µ-Cl)]2 and adding2equiv of PEt3,for afinal P/Rh ratio of2.Under H2,the norbor-nadiene(nbd)is removed by hydrogenation,and we get RhH2Cl(PEt3)2,which is an active hydrogenation catalyst under these conditions.8A key prerequisite for catalysis in many systems is coordinative unsaturation,that is,an open site at the metal.HPEt3H Rh PEt3PEt39.5As predicted by the mechanism of Fig.9.3,the hydrogen gives syn addition to the alkene,although it is possible to tell only this in certain cases.For example,DD(9.3)33DIsomerization is often a minor pathway in a hydrogenation catalyst because the intermediate alkyl mayβ-eliminate before it has a chance to reductively eliminate. The more desirable catalysts,such as9.4,tend to give little isomerization.The selectivity for different alkenes(the hydrogenation rates change in the following order:monosubstituted>disubstituted>trisubstituted>tetrasubstituted=0)is determined by how easily they can bind to the metal;the poorer ligands among them are reduced slowly,if at all.This means that9.4reduces the triene9.6 largely to the octalin9.7(Eq.9.4).Heterogeneous catalysts give none of this product,but only the fully saturated decalin(9.9),and the isomerization product, tetralin(9.8)(Eq.9.4).The C=O and C=N double bonds of ketones and imines are successfully reduced only by certain catalysts.Other functional groups that244HOMOGENEOUS CATALYSIS can be reduced by heterogeneous catalysts,such as−CN,−NO2,−Ph,and −CO2Me are not reduced by the usual homogeneous catalysts.2339.99.89.7isomerizationproduct (minor)hydrogenation product(major)+9.6(9.4)IrCl(PPh3)3,the iridium analog of9.4,is inactive because of the failure of the dihydride IrH2Cl(PPh3)3to lose phosphine;this is a result of the stronger metal–ligand bond strengths usually found for the third-row ing the same general strategy we saw for Rh,[(cod)Ir(µ-Cl)]2is active if only2mol of phosphine are added per metal.A more useful catalyst is obtained by replacing the chloride with a“noncoordinating”anion and changing the ligands to give the precursors[(cod)Ir(PMePh2)2]+PF6−,9.10,and[(cod)Ir(py)(PCy3)]+PF6−, 9.11.8These catalysts tend to bind a solvent,such as EtOH,much morefirmly than do such uncharged catalysts as9.4,for example,to give the isolable species [IrH2(solv)2(PMePh2)2]+PF6−(solv=acetone,ethanol,or water).This results from the presence of hydrides and the net cationic charge,which tend to make the metal a harder Lewis acid.Unlike many noncationic catalysts,these species are also air stable and even tolerate halocarbons.As a result,the catalyst can be used in CH2Cl2,a much more weakly coordinating solvent than pound 9.11has the unusual feature that it can reduce even highly hindered alkenes such as Me2C=CMe2.This is probably because these alkenes do not have to compete with dissociated phosphine or a coordinating solvent for a site on the metal,and perhaps also because the{Ir(py)(PCy3)}+fragment is not very bulky.The high activity of9.10atfirst escaped attention because it was initially tested in EtOH, which at that time was the conventional solvent6for hydrogenation.Screening a new catalyst under a variety of conditions is therefore advisable.9.119.10+Ir PMe2PhPMe2Ph+IrPCy3pyDirecting EffectsThe catalyst 9.11shows strong directing effects,which can be very useful in organic synthetic applications (see Section 14.2).9This means that H 2is added to one face of the substrate,if there is a coordinating group (e.g.,−OH,−COMe,−OMe)on that face (Eq.9.5).The net positive ionic charge makes the metal hard enough to bind to the directing group and,as IrL 2+is a 12e fragment,it still has enough vacant sites left to bind both H 2and the alkene to give the key intermediate 9.12.Of the four possible geometrical isomers of the saturated ketone,only one is formed,H 2having been added cis to the directing group.OO HH3(9.5)Asymmetric CatalysisThe corresponding “RhL 2+”catalysts were developed by Schrock and Osborn.10a Their most important application is asymmetric catalysis.10b Equation 9.6shows how the achiral alkene 9.13can give two enantiomers 9.14and 9.15on hydro-genation.RCCH 2R ′R C CH 3R ′H R C CH 3R ′H 9.159.149.13+(9.6)Any alkene having this property is called prochiral ,which implies that the two faces of the molecule are different.In 9.13,one face has a clockwise arrangement of R,R ,and =CH 2about the central carbon;the other face has an anticlockwise arrangement of these groups.If the H 2is added from one face,one enantiomer is formed;if from the other face,the other enantiomer is the product.If we were to bias the addition of H 2to one face,then we would have an asymmetric synthesis.As shown in Eq.9.7,when a prochiral alkene binds to an achiral metal,two enantiomers are formed;that is,the complex is chiral even though neitherthe ligand nor the metal were chiral before the complex was formed.One way of thinking about this is to regard the carbon indicated by the asterisk as having four different substituents,one of which is the metal.RC CH2 R′RC CH2R′RC CH2R′ML nML n**9.179.16enantiomers+(9.7)RC CH2 R′RC CH2R′RC CH2R′MLML n**diastereomers+9.189.19**n*(9.8)The key point is that if the ML n catalyst fragment can also be made chiral (say because a ligand L has an asymmetric carbon),then we can use one resolved enantiomer of the chiral complex as catalyst.In Eq.9.8,instead of forming two enantiomeric complexes such as9.16and9.17,which react at equal rates to give achiral products,we will have diastereomeric alkene–catalyst complexes, 9.18and9.19,because we now have two asymmetric centers present,C∗in the coordinated alkene and the asymmetric ML∗n fragment.Since diastereomers gen-erally have different chemical properties,9.18and9.19normally have different rates of hydrogenation.This bias on the rates of hydrogenation can selectively give us one of the pair of enantiomers9.14or9.15over the other.In summary, one enantiomer of the catalyst should preferentially give one enantiomer of the hydrogenated alkene,and the other enantiomer give the other product.This is an extremely valuable method because we can obtain a large amount of one enan-tiomeric product from a small amount of resolved material(the catalyst).This is precisely the method Nature uses to make pure enantiomers;enzymes are such efficient asymmetric catalysts that essentially only one enantiomer is normally formed in most enzymatic processes.In asymmetric hydrogenation,95–99%enantiomeric excess[e.e.=100×{amount of major isomer−amount of minor isomer}/{total of both isomers}] can be obtained in favorable cases.Thefirst alkenes to be reduced with high asymmetric induction contained a coordinating group,examples of which are shown as9.20and9.21.PhNHCOMeCO2MeNHCOMeCO2Me9.219.20These are believed to bind to the metal via the amide carbonyl just as we saw hap-pen in directed hydrogenation.This improves the rigidity of the alkene–catalyst complex,which in turn increases the chiral discrimination of the system.As in directed hydrogenation,a12e catalyst fragment,such as that formed from the Schrock–Osborn catalyst,is required.One of the best ways of making the metal chiral is to use the ligand shown as9.22,called BINAP.This ligand has a so-called C2axis;this simply means that it has the symmetry of a helical bolt,which can,of course,either have a left-handed or a right-handed thread.The chiral centers impose a twist on the conformation of the BINAP-metal complex,which in turn leads to a chiral, propeller-like arrangement of the phenyl groups on phosphorus(9.23).These phenyl groups can be thought of as transmitting the chiral information from the asymmetric centers to the binding site for the alkene.The advantage of a C2 symmetry is that the substrate sees the same chirality however it binds;we can think of the substrate as being analogous to a nut with a left-hand thread that will mate with a left-handed(but not a right-handed)bolt,whichever face of the nut is tried.2C2 axis2P PM9.23In the simplest case,one face of the substrate binds better to the catalyst than does the other.Let us say that,if H2were added to this face,we would get the S hydrogenation product.It was once thought that this preferential binding of the substrate always determines the sense of asymmetric induction.Halpern andco-workers 11showed that in a system that gives the R product in good yield,the metal is bound to the “wrong”face in the major diastereomer (9.24),the face that would be expected to give the S product,and so it is the minor isomer of the catalyst–alkene complex that gives rise to most of the product.This in turn means that the minor isomer must react at about 103times the rate of the major isomer (Eq.9.9).Since 9.24and 9.25interconvert rapidly,9.24is continually converting into 9.25because the faster hydrogenation of 9.25continually depletes L n M *L n M *HHL n M *RR ′R ′R RR ′RR ′H R ′R H9.25minor 9.24majorR -product minor S -product major(9.9)the concentration of this minor isomer.Note that Eq.9.9is an example of the “olefin mechanism.”Asymmetric alkene hydrogenation was used in the successful commercial pro-duction of the Parkinson’s disease drug L -DOPA by hydrogenation of the alkene9.26and of the pain reliever,naproxen.12aNHCOMeCO 2MeMeOAcO9.26Another commercial success,this time for Ciba–Geigy,now Novartis,has been the synthesis of the herbicide,(S )-metolachlor,from the imine shown below using an iridium catalyst.The key advantage of iridium is the extremely impres-sive rate (>200,000turnovers h −1)and catalyst lifetime (∼106turnovers)at the。
Some recent advances in the bioprocessing of bauxite

1. Introduction
The use of microorganisms in leaching and beneficiation of lean grade ores and minerals is widely considered an efficient, economical and environmentally benign alternative to conventional hydrometallurgical operations. Biohydrometallurgy is commercially exploited in the recovery of copper and uranium, and is also used for increasing the recovery of finely disseminated gold from refractory ores like pyrite and arsenopyrite ŽRawlings, 1998; Natarajan, 1998.. Bacterial oxidation of sulphide ores using chemoautotrophic bacteria such as Thiobacillus ferrooxidans and Thiobacillus thiooxidans is a well-known process in biohydrometallurgy, and thought to occur by a combination of direct and indirect mechanisms ŽEhrlich, 1992.. These bacteria attach themselves to the sulphide ore particles and form etch patterns on the mineral surface. This is known as direct attack mechanism. Thiobacillus also oxidises ferrous ions in the solution to produce ferric ions, which indirectly oxidises the sulphides. This is called indirect mechanism because the bacterium is not directly involved in the leaching process. Biohydrometallurgy of non-sulphidic minerals has received little attention in the past, compared to bioleaching of sulphidic minerals by Thiobacilli that dominates the literature. There are several examples of such microbe-mineral systems in which selective degradation of mineral componentŽs. have been found to occur through the action of metabolic products, e.g., bioleaching of aluminium from alumino-silicates ŽGroudev et al., 1982., biological removal of iron from sands, kaolins ŽGroudev et al., 1983, 1985., china clay ŽChaudhury and Das, 1990., bioleaching of nickel from laterites ŽBosecker, 1985; McKenzie et al., 1987., and biobeneficiation of bauxite to remove impurities like silica ŽGroudeva and Groudev, 1983., calcium ŽModak et al., 1999., iron ŽDeo et al., 1999., etc. Biobeneficiation differs from bioleaching in that it refers to the selective dissolution of undesirable mineral componentŽs. from the ore by direct or indirect action of the microbes, thereby enriching the desirable mineral content in the ore.
Handbook of Heterogeneous Catalysis
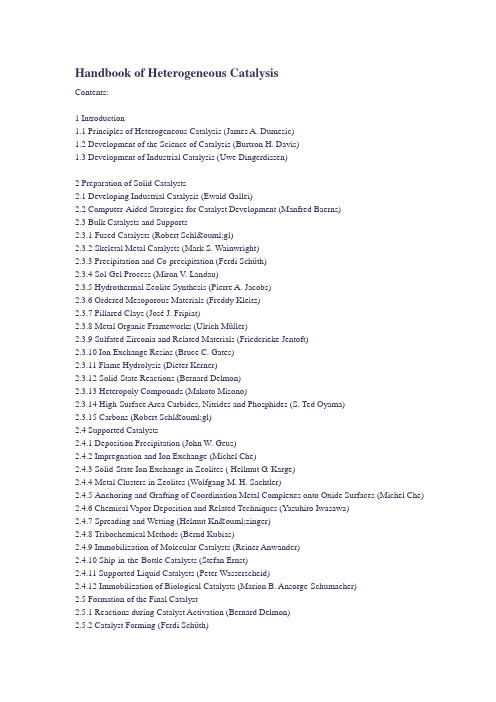
Handbook of Heterogeneous CatalysisContents:1 Introduction1.1 Principles of Heterogeneous Catalysis (James A. Dumesic)1.2 Development of the Science of Catalysis (Burtron H. Davis)1.3 Development of Industrial Catalysis (Uwe Dingerdissen)2 Preparation of Solid Catalysts2.1 Developing Industrial Catalysis (Ewald Gallei)2.2 Computer-Aided Strategies for Catalyst Development (Manfred Baerns)2.3 Bulk Catalysts and Supports2.3.1 Fused Catalysts (Robert Schlögl)2.3.2 Skeletal Metal Catalysts (Mark S. Wainwright)2.3.3 Precipitation and Co-precipitation (Ferdi Schüth)2.3.4 Sol-Gel Process (Miron V. Landau)2.3.5 Hydrothermal Zeolite Synthesis (Pierre A. Jacobs)2.3.6 Ordered Mesoporous Materials (Freddy Kleitz)2.3.7 Pillared Clays (José J. Fripiat)2.3.8 Metal Organic Frameworks (Ulrich Müller)2.3.9 Sulfated Zirconia and Related Materials (Friederieke Jentoft)2.3.10 Ion Exchange Resins (Bruce C. Gates)2.3.11 Flame Hydrolysis (Dieter Kerner)2.3.12 Solid-State Reactions (Bernard Delmon)2.3.13 Heteropoly Compounds (Makoto Misono)2.3.14 High-Surface Area Carbides, Nitrides and Phosphides (S. Ted Oyama)2.3.15 Carbons (Robert Schlögl)2.4 Supported Catalysts2.4.1 Deposition Precipitation (John W. Geus)2.4.2 Impregnation and Ion Exchange (Michel Che)2.4.3 Solid-State Ion Exchange in Zeolites ( Hellmut G. Karge)2.4.4 Metal Clusters in Zeolites (Wolfgang M. H. Sachtler)2.4.5 Anchoring and Grafting of Coordination Metal Complexes onto Oxide Surfaces (Michel Che) 2.4.6 Chemical Vapor Deposition and Related Techniques (Yasuhiro Iwasawa)2.4.7 Spreading and Wetting (Helmut Knözinger)2.4.8 Tribochemical Methods (Bernd Kubias)2.4.9 Immobilization of Molecular Catalysts (Reiner Anwander)2.4.10 Ship-in-the-Bottle Catalysts (Stefan Ernst)2.4.11 Supported Liquid Catalysts (Peter Wasserscheid)2.4.12 Immobilization of Biological Catalysts (Marion B. Ansorge-Schumacher)2.5 Formation of the Final Catalyst2.5.1 Reactions during Catalyst Activation (Bernard Delmon)2.5.2 Catalyst Forming (Ferdi Schüth)2.6 Standard Catalysts2.6.1 Non-Zeolitic Standard Catalysts (Geoffrey C. Bond)2.6.2 Zeolite Standard Catalysts (Michael Stöcker)3 Characterization of Solid Catalysts3.1 Physical Properties3.1.1 Surface Area and Porosity (Kenneth S. W. Sing)3.1.2 Particle Size and Dispersion Measurements (Pierre Gallezot)3.1.3 Structure and Morphology X-Ray Powder Diffraction (Daniel Herein)3.1.3.2 X-Ray Absorption Spectroscopy (Diek C. Koningsberger)3.1.3.3 Electron Microscopy and Diffraction (Sbhaya K. Datye)3.1.3.4 Scanning Probe Techniques (Flemming Besenbacher)3.1.3.5 Heterogeneous Catalysis and High Electric Fields (Norbert Kruse)3.1.3.6 Gamma Spectroscopy (J. W. Niemantsverdriet)3.1.3.7 Solid-State NMR Spectroscopy (Michael Hunger)3.1.3.8 Vibrational Spectroscopy (Helmut Knözinger)3.1.3.9 Neutron Scattering (Hervé Jobic)3.1.3.10 Morphological Characteristics (Friedrich Schmidt)3.1.4 Mechanical Properties (Friedrich Schmidt)3.2 Chemical Properties3.2.1 Bulk Chemical Composition (Peter Albers)3.2.2 Surface Chemical Composition (Edmund Taglauer)3.2.3 Valence States3.2.3.1 Photoelectron and Auger Electron Spectroscopy (Giuliano Moretti)3.2.3.2 UV/Vis and EPR Spectroscopy (Michel Che)3.2.3.3 Photoluminescence Spectroscopy (Masakazu Anpo)3.2.3.4 Muon Spin Resonance (Emil Roduner)3.2.3.5 Temperature-Programmed Reduction and Oxidation (Helmut Knözinger)3.2.4 Acidity and Basicity3.2.4.1 Concepts and Analysis of Surface Acidity and Basicity (Hellmut G. Karge)3.2.4.2 Thermochemical Characterization of Surface Acidity and Basicity (James A. Dumesic) 3.2.4.3 IR Spectroscopy for the Characterization of Surface Acidity and Basicity (Helmut Knözinger)3.2.4.4 NMR Spectroscopy for the Characterization of Surface Acidity and Basicity (Michael Hunger)3.2.5 Active Phase-Support Interactions3.2.5.1 Metal-Support Interactions (Bert M. Weckhuysen)3.2.5.2 Oxide-Oxide Interactions (Bert M. Weckhuysen)3.2.6 Carbonaceous Deposits (Peter Albers)3.3 IUPAC Recommandations3.3.1 Reporting Physisorption Data for Gas/Solid Systems3.3.2 Methods and Procedures for Catalyst Characterization (J. Haber, J. H. Block, B. Delmon)4 Model Systems4.1 Single-Crystal Surfaces (Gabor A. Somorjai)4.2 Supported Metal Clusters (Bruce C. Gates)4.3 Metallic Glasses (Alfons Baiker)4.4 Bimetallic Model Catalysts (José A. Rodriguez)4.5 Ultrathin Oxide Films (Hans-Joachim Freund)4.6 Oxide Model Systems (Hicham Idriss)4.7 Microcrystalline Oxides (Adriano Zecchina)4.8 Oxide Solid Solutions (Frank S. Stone)5 Elementary Steps and Mechanisms5.1 Chemisorption5.1.1 Principles of Chemisorption (Hans-Joachim Freund)5.1.2 Reactivity Index Relations in Theoretical Heterogeneous Catalysis (Rutger van Santen)5.2 Microkinetics5.2.1 Rates of Catalytic Reactions (James A. Dumesic)5.2.2 Dynamics of Surface Reactions (G. Ertl)5.2.3 Theoretical Modelling of Catalytic Reactions (Jens K. Nørskov)5.2.4 Non-linear Dynamics: Oscillatory Kinetics and Spatio-Temporal Pattern Formation (Gerhard Ertl)5.2.5 Isotopic Labeling and Kinetic Isotope Effects (Frank Bauer)5.2.6 Transient Catalytic Studies (Kai-Olaf Hinrichsen)5.3 Factors Influencing Catalytic Action5.3.1 Substituent Effects (Helmut Knözinger)5.3.2 Spillover Effects (Frank Rößner)5.3.3 Ensemble and Ligand Effects in Metal Catalysis (Wolfgang M. H. Sachtler)5.3.4 Promotors and Poisons (Bruce E. Koel)5.4 Hydrocarbon Reaction Mechanisms (Bruce C. Gates)5.5 Computer Simulations5.5.1 Computer Simulations of Structures and Reactivity (C. Richard A. Catlow)5.5.2 Molecular Simulation of Adsorption and Diffusion (Doros N. Theodorou)5.5.3 Computer Simulation of Shape Selectivity Effects (Berend Smit)6 Makrokinetics and Transport Processes6.1 Rate Procurement and Kinetic Modelling (Jacob A. Moulijn)6.2 Diffusion Coefficients in Porous Media (Jörg Kärger)6.3 Simultaneous Heat and Mass Transfer and Chemical Reaction (Gerhard Emig)6.4 NMR Tomography (Lynn Gladden)6.5 Positron Emitters in Catalysis Research (Gert Jonkers)6.6 Computational Fluid Dynamics Simulation of Catalytic Reactors (Olaf Deutschmann)7 Activity Loss7.1 Deactivation and Regeneration (Jacob A. Moulijn)7.2 Recycling of Spent Catalysts7.2.1 Recycling of Spent Catalysts Containing Precious Metals (Christian Hagelüken)7.2.2 Recycling of Spent Catalysts Containing Base Metals (Sean Axon)8 Special Catalytic Systems8.1 Electrocatalysis8.1.1 Fundamentals of Electrocatalysis (Katharina Krischer)8.1.2 Electrochemical Modification of Catalytic Activity (Costas G. Vayenas)8.1.3 Industrial Electrocatalysis (Sigmar Bräuninger)8.2 Photocatalysis (M. Anpo)8.3 Chemical Sensors Based on Catalytic Reactions (Klaus Schierbaum)8.4 Heterogeneous Catalysis in Non-Conventional Solvents (Roger Gläser) 8.5 Sonocatalysis (Kenneth S. Suslick)9 Laboratory Testing of Solid Catalysts9.1 Laboratory Catalytic Reactors: Aspects of Catalyst Testing (Jacob A. Moulijn) 9.2 Ancillary Techniques in Laboratory Units for Catalyst Testing (Jens Weitkamp) 9.3 High-Throughput-Experimentation in Heterogeneous Catalysis (Ferdi Schüth)10 Reaction Engineering10.1 Catalytic Fixed-Bed Reactors (Gerhart Eigenberger)10.2 Fluidized-Bed Reactors (Joachim Werther)10.3 Slurry Reactors (Adrian Schumpe)10.4 Unsteady-State Reactor Operation (Eng. Yurii Matros)10.5 Short-Contact-Time Reactors (Götz Veser)10.6 Catalytic Distillation (Domenico Sanfilippo)10.7 Membrane Reactors (Jürgen Caro)10.8 Microstructured Catalytic Reactors (Albert Renken)11 Environmental Catalysis11.1 The Role of Catalysis in Environmental Protection (Wilhelm Keim)11.2 Automotive Exhaust Treatment (Egbert Lox)11.3 Treatment of Flue Gases from Stationary Sources (Pär Gabrielsson) 11.4 Catalytic Dehalogenation (R. F. Howe)11.5 Removal of Volatile Organic Compounds (James J. Spivey)11.6 Catalytic Combustion (Pio Forzatti)11.7 Catalytic Routes to Hydro(chloro)fluorocarbons (Z. Ainbinder)11.8 Heterogeneous Catalysis in the Troposphere (Valentin M. Parmon)11.9 Conversion of Biomass on Solid Catalysts (Pierre Gallezot)11.10 Catalysis in Water Remediation (Klaus-Dieter V orlop)12 Inorganic Reactions12.1 Ammonia Synthesis (Robert Schlögl)12.2 Ammonia Oxidation (Stephan T. Hatscher)12.3 HCN Production (Jörg Sauer)12.4 Claus Process (Eckhard Jüngst)12.5 Oxidation of Sulfur Dioxide (Fritz Näumann)Müller-Rochow Synthesis (Wilfried Kalchauer)12.7 Hydrazine Decomposition (Wilhelm Keim)13 Energy-Related Catalysis13.1 Perspectives in Oil Refining (Colin Baudouin)13.2 Hydrotreating (Roel Prins)13.3 Hydrodemetalation13.4 Catalytic Reforming (Mark D. Moser, Paula L. Bogdan)13.5 Fluid Catalytic Cracking (E. Thomas Habib, jr.)13.6 Hydrocracking and Dewaxing (J. A. Rob van Veen)13.7 Isomerization (S. T. Sie)13.8 Alkylation of Isobutane (Jens Weitkamp)13.9 Oligomerization of Alkenes (Cril T. O'Connor)13.10 Etherification (Outi Krause)13.11 Steam Reforming (Jens Rostrup-Nielsen)13.12 Water Gas Shift (Martin Muhler)13.13 Methanol Synthesis (John Bogild Hansen)13.14 Methanol to Hydrocarbons (Steinar Kvisle)13.15 Fischer-Tropsch Synthesis (Mark E. Dry)13.16 Gas-to-Liquids (Arend Hoek)13.17 Oxidative Coupling of Methane (Manfred Baerns)13.18 Catalysis in Direct Coal Hydrogenation (Matthias W. Haenel)13.19 Catalysis in Coal and Carbon Gasification (Ljubisa R. Radovic)13.20 Fuel Cell-Related Catalysis13.20.1 Fuel Processors (Ralf Peters)13.20.2 Fuel Cells (Jürgen Garche)14 Organic Reactions14.1 Aromatization of Light Alkanes (Cyril T. O'Connor)14.2 Ring Opening of Aromatics (Jens Weitkamp)14.3 Alkylation of Aromatics (David L. Stern)14.4 Isomerization and Transalkylation of Alkylaromatics (Jeffrey S. Beck)14.5 Non-Oxidative Activation of Alkanes (Yvonne Traa)14.6 Dehydrogenation of Alkanes (Karl J. Caspary)14.7 Dehydrogenation of Ethylbenzene (Martin Muhler)14.8 Metathesis of Alkenes (J. C. Mol)14.9 Dehydrogenation of Alcohols: Formaldehyde (Wei-Lin Dai)14.10 Hydrogenation Reactions14.10.1 Selective Hydrogenation of Hydrocarbons (Johann Gaube)14.10.2 Selective Hydrogenation of Functionalized Hydrocarbons (Hans-Ulrich Blaser) 14.10.3 Regioselective Hydrogenations (Peter Claus)14.10.4 Oleochemical Reactions (Bernhard Gutsche)14.11 Selective Oxidations14.11.1 Fundamentals of Hydrocarbon Oxidation (J. Haber)14.11.2 Oxidative Dehydrogenation of Alkanes (Anders Holmen)14.11.3 Oxyfunctionalization of Alkanes (Stephan Schunk)14.11.4 Oxyfunctionalization of Alkyl Aromatics (Frank Rosowski)14.11.5 Direct Ring Oxidation of Aromatics to Phenols (Elias Klemm)14.11.6 Heterogeneous Catalysis of Alkene Epoxidation (Mark A. Barteau)14.11.7 Oxyacetylation: Vinyl Acetate from Ethene (Stephan A. Schunk)14.11.8 Oxidation of C3 Hydrocarbons14.11.9 Ammoxidation of Hydrocarbons (Robert C. Grasselli)14.11.10 Ammoximation (Robert C. Grasselli)14.11.11 Oxidation of Alcohols (Alfons Baiker)14.11.12 Phenol Hydroxylation and Related Oxidations (Giuseppe Bellussi)14.12 Amination Reactions (Alfons Baiker)14.13 Halogenation Reactions (Ratnasamy)14.14 Acylation of Aromatics (Herman van Bekkum)14.15 Elimination and Addition Reactions (Helmut Knözinger)14.16 Stereoselective Reactions14.16.1 Catalysis on Chiral Surfaces (Alfons Baiker)14.16.2 Enantioselective Catalysis on Anchored Chiral Complexes (Hans-Ulrich Blaser) 14.16.3 Diastereoselective Catalysis (Michèle Besson)14.17 Miscellaneous Catalytic Systems14.17.1 Hydroformylation on Solid Catalysts (Vladimir A. Likholobov)14.17.2 Wacker Chemistry with Solid Catalysts (Pierre A. Jacobs)14.17.3 Oxidations on Immobilized Molecular Catalysis (Dirk De V os)14.17.4 Production of Chemicals from Carbon Dioxide (Paul Ratnasamy)15 Polymerization Reactions15.1 Polymerization on Phillips-Type Catalysts (Max P. McDaniel)15.2 Polymerization on Molecular Catalysts (Gerhard Fink)16 Reactions on Immobilized Biocatalysts (Udo Kragl)。
Organocatalysis – Catalysts and Applications
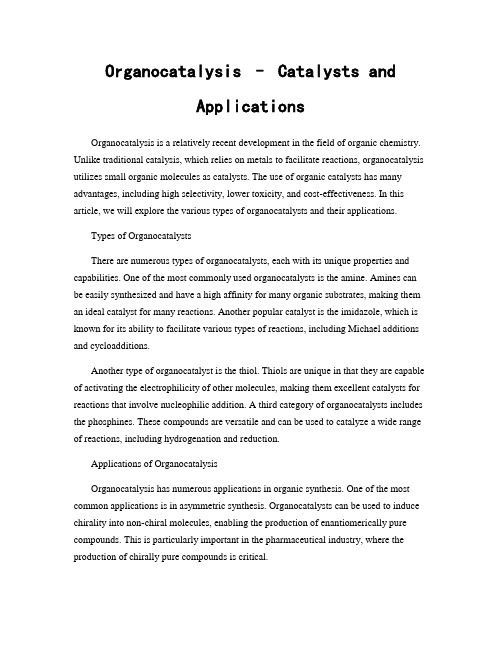
Organocatalysis – Catalysts andApplicationsOrganocatalysis is a relatively recent development in the field of organic chemistry. Unlike traditional catalysis, which relies on metals to facilitate reactions, organocatalysis utilizes small organic molecules as catalysts. The use of organic catalysts has many advantages, including high selectivity, lower toxicity, and cost-effectiveness. In this article, we will explore the various types of organocatalysts and their applications.Types of OrganocatalystsThere are numerous types of organocatalysts, each with its unique properties and capabilities. One of the most commonly used organocatalysts is the amine. Amines can be easily synthesized and have a high affinity for many organic substrates, making them an ideal catalyst for many reactions. Another popular catalyst is the imidazole, which is known for its ability to facilitate various types of reactions, including Michael additions and cycloadditions.Another type of organocatalyst is the thiol. Thiols are unique in that they are capable of activating the electrophilicity of other molecules, making them excellent catalysts for reactions that involve nucleophilic addition. A third category of organocatalysts includes the phosphines. These compounds are versatile and can be used to catalyze a wide range of reactions, including hydrogenation and reduction.Applications of OrganocatalysisOrganocatalysis has numerous applications in organic synthesis. One of the most common applications is in asymmetric synthesis. Organocatalysts can be used to induce chirality into non-chiral molecules, enabling the production of enantiomerically pure compounds. This is particularly important in the pharmaceutical industry, where the production of chirally pure compounds is critical.Organocatalysts are also used in the synthesis of natural products. Many natural products have complex structures that are difficult to synthesize using traditional methods. Organocatalysis offers an alternative approach that can facilitate the synthesis of complex natural products. Additionally, organocatalysis is increasingly being used in polymerization reactions, where it can enhance the selectivity and efficiency of the reaction.ConclusionOrganocatalysis has emerged as an important field of research in organic chemistry. The use of organic catalysts has significant benefits over traditional metal catalysts, including high selectivity, lower toxicity, and cost-effectiveness. Organocatalysts are capable of facilitating a wide range of reactions, including asymmetric synthesis, natural product synthesis, and polymerization reactions. As research in this field continues to evolve, we can expect to see even more applications and advances in organocatalysis.。
催化10
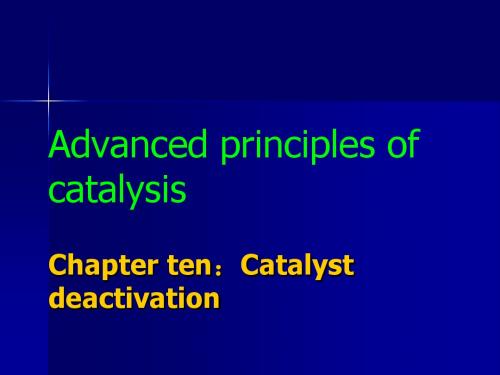
Theoretical approaches
– – – – Surface Brownian motion Migration rates depend on T and atmosphere Smaller crystals have higher vapor pressure Transport over surface and through gas phase
Loss of mechanical strength
– Often assisted by thermal and chemical effects – Instances
Steam reforming
– Loss of silicate
AluminaAlumina-based cats
– Sulfate reacts with alumina
Not surface poisoning, but bulk compound formation
– Easily identified by XRD
Cases of Deactivation
Sintering
– Favored in catalyst cases
Extremely small crystallite size, porous supports, reactive atmosphere, relatively lower temperature Surface and bulk atoms become mobile at temperatures of about one-third and one-half of the melting point oneoneNanosized particles is far more mobile than bulk solids Progressive steps in sintering
07-均相催化
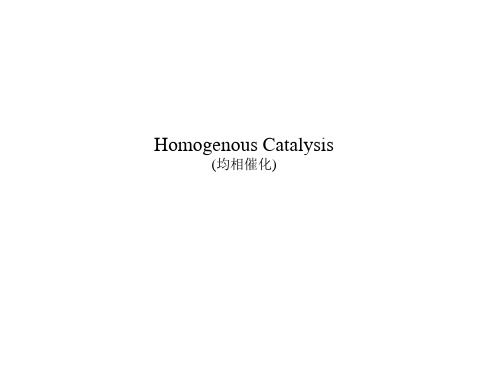
5
Over 1,000,000 tons of acetic acid are produced every year using the Monsanto process. The reaction is highly selective, goes in high yield, and is extremely fast. Adv. Organomet. Chem. 1979, 17, 255
Q5: Electron Counting on the following organometallics?
2.1 ALKENE HYDOGENATION (烯烃氢化)
The way each type activates H2) oxidative addition, (2) heterolytic activation,and (3) homolyticactivation. A key prerequisite for catalysis is coordinative unsaturation, an open site at the metal.
Q1. Which of the following will be more reactive towards oxidative addition of dihydrogen? A. [Co(dppe)2]+ B. [Ir(dppe)2]+
Q2. Which of the following will be more reactive towards oxidative addition of dihydrogen? A. Rh(PPh3)3Cl B. Rh(PPh3)2(CO)Cl
Dewar-Chatt-Duncanson model
- 1、下载文档前请自行甄别文档内容的完整性,平台不提供额外的编辑、内容补充、找答案等附加服务。
- 2、"仅部分预览"的文档,不可在线预览部分如存在完整性等问题,可反馈申请退款(可完整预览的文档不适用该条件!)。
- 3、如文档侵犯您的权益,请联系客服反馈,我们会尽快为您处理(人工客服工作时间:9:00-18:30)。
A brief introduction to OrganocatalysisJi GuojingMay. 25th,2013Contents1 introduction2enamine catalysisi t l iy3 iminium catalysis4 Hydrogen‐bond activation5 Carbene catalysisactivation modes6 other7 summary and outlookintroductionOrganocatalysis is the acceleration of chemical reactions with a substoichiometric g y amount of an organic compound which does not cotain a metal atom.1970s Hajos L ‐proline2000 List and MacMillanIn the early 20th century ‐1960s Pracejus alkaloidj p Bredig Cinchona alkaloidUsed for enantioselective construction of C ‐C, N C O C S bondsintroductionInherent benefits C ‐N, ‐O, ‐S, C ‐P , C ‐halogen fInsensitive to mositure and air Inexpensive‐Readily avaliable from bio material Operationally easy to handleNon ‐toxic ,easily removed from waste streams Rich, new avenue for academic thought ,gExamples: Proline, cinchona alkaloids or other secondary amine derivativesOrganocatalytic Activation modesintroductionNNOMeN N S O P O R NHO 2CPhRXH HYO OH RMeRIminium F F Hydrogen Bonding Hydrogen BondingEnamineO -R 3NRS MeMeR N ROH OMeN N N FF F AmmoniumYlide NPhase TransferO CarbeneEnamine catalysisE i l iPeter, I. D.; Lionel, M., Angew. Chem. Int. Ed. 2004, 43, 5138.E i l iAld l tiEnamine catalysis Aldol reaction MacMillan, D. et al. J. Am. Chem. Soc. 2002, 124, 6798.Jorgensen, K. A . et al. mun . 2002, 620.E i l iMannich reactionEnamine catalysis List, B.; Pojarliev, P . et al. J. Am. Chem. Soc . 2002,124, 827.Wrdova, A. C.; Barbas III, C. F., Tetrahedron Lett. 2002, 43, 7749.E i l iProposed transition statesEnamine catalysis p List, B. et al. J. Am.Chem. Soc . 2003, 125, 2475.Dalko, P . I.; Moisan, L., Angew. Chem. Int . Ed . 2004, 43, 5138.E i l iEnamine catalysis ‐Ketone Aldehyde AldolAldolizationAdditions to EnonesList, B. et al. J. Am. Chem. Soc . 2002, 2395.List, B. et al. Angew. Chem. Int. Ed . 2003, 2758.Additons to NitroalkenesBarbas III, C. et al. Org. Lett . 2004, 2527.List, B. et al. Angew. Chem. Int. Ed . 2004, 3958.E i l iα‐Aminooxylation of aldehydes Enamine catalysis y yMacMillan, D . et al. J. Am. Chem. Soc . 2003, 125, 10808.S 2 Alkylation N yList, B. et al. J. Am. Chem. Soc . 2003, 125, 450.Enamine catalysisE i l iJorgensen, K. A. et al. J. Am. Chem. Soc. 2009, 131, 7153.E i l iAsymmetric alkylation of aldehyded Trifluoride of aldehyded Enamine catalysis y y y yMacMillan, D . et al. Science , 2008, 322, 77.MacMillan, D. et al. J. Am. Chem. Soc . 2009, 131, 10875.E i l iMichael addition / gold ‐catalyzed reactionEnamine catalysis Belot, S.; Vogt, K. A.; Besnard, C . et al. Angew. Chem. Int. Ed . 2009, 48, 8923.Contents1 introduction2enamine catalysisi t l iy3 iminium catalysis4 Hydrogen‐bond activation5 Carbene catalysisactivation modes6 other7 summary and outlookiminiums catalysisi i i l iMukaiyama‐MichealMacMillan, D. et al. J. Am. Chem. Soc. 2003,125, 1192.i i i l iMalonate additoniminiums catalysis Jorgensen, K . A. et al. Angew. Chem. Int. Ed . 2003, 42, 661.Dicarbonyl additonsJorgensen, K . A. et al. Angew. Chem. Int. Ed . 2003, 42, 4955.iminiums catalysisi i i l iDiels‐Alder reactionsMacMillan, D. et al. J. Am. Chem. Soc. 2000, 122, 4243. Thiol additionsDeng, L. et al. Angew. Chem. Int. Ed. 2002, 41, 338.i i i l iiminiumscatalysis Friedel ‐Crafts Alkylation y[43]l dditi MacMillan, D. et al. J. Am. Chem. Soc . 2001, 123, 4370.[4+3]cycloadditionsHarmata, M. et al. J. Am. Chem. Soc . 2003, 125, 2058.ii i l i Domino reactioniminiums catalysis Jorgensen, K. A . et al. J. Am. Chem. Soc . 2006, 128, 14986.Contents1 introduction2enamine catalysisi t l iy3 iminium catalysis4 Hydrogen‐bond activation5 Carbene catalysisactivation modes6 other7 summary and outlookHydrogen-bond activationH d b d i iThe and imine activation mode of carbonylThe activation mode of C=C by H‐bondsHydrogen-bond activationH d b d i iMannich reactionJacobsen, E. et al. J. Am. Chem. Soc. 2002, 124, 12964. Micheal additionsMi h l dditiTokemoto, Y. et al. J. Am. Chem. Soc. 2003, 125, 12672.H d b d i iN l hili dditi t b i iHydrogen-bond activationNuclephilic additions to oxocarbenium ionsJacobsen, E. et al. J. Am. Chem. Soc. 2008, 7198.H d b d i i Asymmetic ene reactionHydrogen-bond activation y Dixon, D . et al. J. Am. Chem. Soc . 2009, 131, 9140.H d b d i iHydrogen-bond activationMannich reactionsTerada, M. et al. J. Am. Chem. Soc. 2004, 126, 5356. Diels‐AlderTerada, M. et al. J. Am. Chem. Soc. 2009, 131, 12882.H d b d i i Aza ‐Cope rerrangementHydrogen-bond activation Rueping, M.; Antonchick, A. P . Angew. Chem. Int. Ed . 2008, 47, 10090.Hd b d i i component Hydrogen-bond activation Three reactionHu, W. H.; Xu, X. F.; Zhou. J . J. Am. Chem. Soc . 2008, 130, 7782.Contents1 introduction2enamine catalysisi t l iy3 iminium catalysis4 Hydrogen‐bond activation5 Carbene catalysisactivation modes6 other7 summary and outlookCarbene catalysisC b l iSila‐Stetter reactionScheidt, K. et al, J. Am. Chem. Soc. 2004, 126, 2314.C b l iTransesterificationCarbene catalysis Schmidt, M. et al. Org. Lett . 2005, 7, 2453.C b l i[4+2] reactionCarbene catalysis []Ye, S.; Huang, X. L. et al. Angew. Chem. Int. Ed . 2009, 48, 192.h i i dother activation modesPhase‐transfer catalysisAndrews, B. I.; Lygo, B. et al. Acc. Chem. Res. 2004, 37, 518.h i i dother activation modesYlide catalysisMetzner, P. et al. J. Org. Chem. 2001, 66, 5620.h i i d other activation modesPhosphoramidesPyridine N‐OxidesSulfoxidesS d l k Summary and outlookEnamine catalysisIminium catalysisHydrogen-bond activationy gCarbene catalysisOther activation modesAcknowledgementProf. Jianbo Wang , Prof. Yan Zhang and Prof. Ming Li All members in laboratory especially Xi WangAll for your attentionf y。