K-band Spectroscopy of Clusters in NGC 40384039
Height and gradient from shading
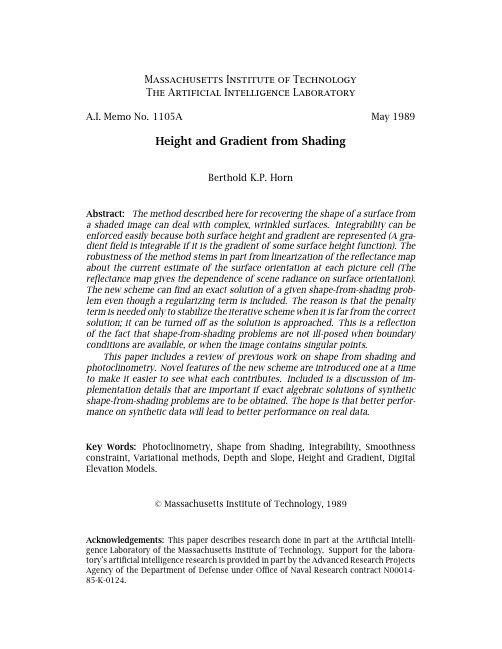
Massachusetts Institute of TechnologyThe Artificial Intelligence LaboratoryA.I.Memo No.1105A May1989Height and Gradient from ShadingBerthold K.P.HornAbstract:The method described here for recovering the shape of a surface from a shaded image can deal with complex,wrinkled surfaces.Integrability can be enforced easily because both surface height and gradient are represented(A gra-dientfield is integrable if it is the gradient of some surface height function).The robustness of the method stems in part from linearization of the reflectance map about the current estimate of the surface orientation at each picture cell(The reflectance map gives the dependence of scene radiance on surface orientation). The new scheme canfind an exact solution of a given shape-from-shading prob-lem even though a regularizing term is included.The reason is that the penalty term is needed only to stabilize the iterative scheme when it is far from the correct solution;it can be turned offas the solution is approached.This is a reflection of the fact that shape-from-shading problems are not ill-posed when boundary conditions are available,or when the image contains singular points.This paper includes a review of previous work on shape from shading and photoclinometry.Novel features of the new scheme are introduced one at a time to make it easier to see what each contributes.Included is a discussion of im-plementation details that are important if exact algebraic solutions of synthetic shape-from-shading problems are to be obtained.The hope is that better perfor-mance on synthetic data will lead to better performance on real data.Key Words:Photoclinometry,Shape from Shading,Integrability,Smoothness constraint,Variational methods,Depth and Slope,Height and Gradient,Digital Elevation Models.©Massachusetts Institute of Technology,1989 Acknowledgements:This paper describes research done in part at the Artificial Intelli-gence Laboratory of the Massachusetts Institute of Technology.Support for the labora-tory’s artificial intelligence research is provided in part by the Advanced Research Projects Agency of the Department of Defense under Office of Naval Research contract N00014-85-K-0124.1.BackgroundThefirst method developed for solving a shape-from-shading problem was restricted to surfaces with special reflecting properties[Rindfleisch66]. For the surfaces that Rindfleisch considered,profiles of the solution can be obtained by integrating along predetermined straight lines in the im-age.The general problem was formulated and solved later[Horn70, 75],using the method of characteristic strip expansion[Garabedian64] [John78]applied to the nonlinearfirst-order partial differential image ir-radiance equation.When the light sources and the viewer are far away from the scene being viewed,use of the reflectance map makes the anal-ysis of shape-from-shading algorithms much easier[Horn77][Horn& Sjoberg79].Several iterative schemes,mostly based on minimization of some functional containing an integral of the brightness error,arose later[Woodham77][Strat79][Ikeuchi&Horn81][Kirk84,87][Brooks& Horn85][Horn&Brooks86][Frankot&Chellappa88].The new method presented here was developed in part as a response to recent attention to the question of integrability1[Horn&Brooks86] [Frankot&Chellappa88]and exploits the idea of a coupled system of equations for depth and slope[Harris86,87][Horn88].It borrows from well-known variational approaches to the problem[Ikeuchi&Horn81] [Brooks&Horn85]and an existing least-squares method for estimat-ing surface shape given a needle diagram(see[Ikeuchi84],chapter11 in[Horn86],and[Horn&Brooks86]).For one choice of parameters,the new method becomes similar to one of thefirst iterative methods ever developed for shape from shading on a regular grid[Strat79],while it degenerates into another well-known method[Ikeuchi&Horn81]for a different choice of parameters.If the brightness error term is dropped, then it becomes a surface interpolation method[Harris86,87].The com-putational effort grows rapidly with image size,so the new method can benefit from proper multigrid implementation[Brandt77,80,84][Brandt &Dinar79][Hackbush85][Hackbush&Trottenberg82],as can existing iterative shape-from-shading schemes[Terzopolous83,84][Kirk84,87]. Alternatively,one can apply so-called direct methods for solving Poisson’s equations[Simchony,Chellappa&Shao89].Experiments indicate that linear expansion of the reflectance map about the current estimate of the surface gradient leads to more rapid convergence.More importantly,this modification often allows the scheme to converge when simpler schemes diverge,or get stuck in local minima of the functional.Most existing iterative shape-from-shading methods1A gradientfield is integrable if it is the gradient of some surface height function.2Height and Gradient from Shadinghandle only relatively simple surfaces and so could benefit from a retrofit of this idea.The new scheme was tested on a number of synthetic images of in-creasing complexity,including some generated from digital terrain mod-els of steep,wrinkled surfaces,such as a glacial cirque with numerous gullies.Shown in Figure1(a)is a shaded view of a digital terrain model, with lighting from the Northwest.This is the input provided to the algo-rithm.The underlying231×178digital terrain model was constructed from a detailed contour map,shown in Figure2,of Huntington ravine on the eastern slopes of Mount Washington in the White Mountains of New Hampshire2.Shown in Figure1(b)is a shaded view of the same digital terrain model with lighting from the Northeast.This is not available to the algorithm,but is shown here to make apparent features of the sur-face that may not stand out as well in the other shaded view.Figure1(c) shows a shaded view of the surface reconstructed by the algorithm,with lighting from the Northwest—it matches Figure1(a)exactly.More impor-tantly,the shaded view of the reconstructed surface with lighting from the Northeast,shown in Figure1(d),matches Figure1(b)exactly also3.With proper boundary conditions,the new scheme recovers surface orientation exactly when presented with noise-free synthetic scenes4.Pre-vious iterative schemes do notfind the exact solution,and in fact wander away from the correct solution when it is used as the initial guess.To ob-tain exact algebraic solutions,several details of the implementation have to be carefully thought through,as discussed in section6.Simple sur-faces are easier to process—with good results even when several of the implementation choices are not made in an optimal way.Similarly,these details perhaps may be of lesser importance for real images,where other error sources could dominate.In the next few sections we review image formation and other elemen-tary ideas underlying the usual formulation of the shape-from-shading problem.Photoclinometry is also briefly reviewed for the benefit of re-searchers in machine vision who may not be familiar with thisfield.We then discuss both the original and the variational approach to the shape-2The gullies are steep enough to be of interest to ice-climbers.3For additional examples of reconstructions from shaded images,see section7. 4In the examples tried,the algorithm always recovered the underlying surface orientation exactly at every picture cell,starting from a random surface ori-entationfield,provided that boundary information was available.Since the question of uniqueness of solutions has not been totally resolved,one cannot be quite certain that there may not be cases where a different solution might be found that happens to alsofit the given image data exactly.1.Background3Figure1.Reconstruction of surface from shaded image.See text.4Height and Gradient from ShadingFigure2.Contour map from which the digital terrain model used tosynthesize Figures1(a)and(b)was interpolated.The surface was mod-eled as a thin plate constrained to pass through the contours at thespecified elevations.The interpolating surface was found by solvingthe biharmonic equation,as described at the end of section5.4.from-shading problem.Readers familiar with the basic concepts may wish to skip over this material and go directly to section5,where the new scheme is derived.For additional details see chapters10and11in Robot Vision[Horn86]and the collection of papers,Shape from Shading[Horn &Brooks89].2.Review of Problem Formulation2.1Image Projection and Image IrradianceFor many problems in machine vision it is convenient to use a camera-2.Review of Problem Formulation5centered coordinate system with the origin at the center of projection and the Z-axis aligned with the optical axis(the perpendicular from the center of projection to the image plane)5.We can align the X-and Y-axes with the image plane x-and y-axes.Let the principal distance(that is,the perpendicular distance from the center of projection to the image plane) be f,and let the image plane be reflected through the center of projection so that we avoid sign reversal of the coordinates.Then the perspective projection equations arex=f XZand y=fYZ.(1)The shape-from-shading problem is simplified if we assume that the depth range is small compared with the distance of the scene from the viewer (which is often the case when we have a narrowfield of view,that is,when we use a telephoto lens).Then we havex≈fZ0X and y≈fZ0Y,(2)for some constant Z0,so that the projection is approximately orthographic. In this case it is convenient to rescale the image coordinates so that we can write x=X and y=Y.For work on shape from shading it is also convenient to use z,height above some reference plane perpendicular to the optical axis,rather than the distance measured along the optical axis from the center of projection.If we ignore vignetting and other imaging system defects,then im-age irradiance E at the point(x,y)is related to scene radiance L at the corresponding point in the scene by[Horn86]E=L π4df2cos4α,(3)where d is the diameter of the lens aperture,f is the principal distance, and the off-axis angleαis given bytanα=1fx2+y2.(4)Accordingly,image irradiance6is a multiple of the scene radiance,with the factor of proportionality depending inversely on the square of the f-5In photoclinometry it is customary to use an object-centered coordinate system.This is because surface shape can be computed along profiles only when strong additional constraint is provided,and such constraints are best expressed in an object-centered coordinate system.Working in an object-centered coordinate system,however,makes the formulation of the shape-from-shading problem considerably more complex(see,for example,[Rindfleisch66]).6Grey-levels are quantized estimates of image irradiance.6Height and Gradient from Shadingnumber7.If we have a narrowfield of view,the dependence on the off-axis angleαcan be neglected.Alternatively,we can normalize the image by dividing the observed image irradiance by cos4α(or whatever the actual vignetting function happens to be).We conclude from the above that what we measure in the image is directly proportional to scene radiance,which in turn depends on(a)the strength and distribution of illumination sources,(b)the surface micro-structure and(c)surface orientation.In order to be able to solve the shape from shading problem from a single image we must assume that the surface is uniform in its reflecting properties.If we also assume that the light sources are far away,then the irradiance of different parts of the scene will be approximately the same and the incident direction may be taken as constant.Finally,if we assume that the viewer is far away,then the direction to the viewer will be roughly the same for all points in the scene.Given the above,wefind that scene radiance does not depend on the position in space of a surface patch,only on its orientation.2.2Specifying Surface OrientationMethods for recovering shape from shading depend on assumptions about the continuity of surface height and its partial derivatives.First of all, since shading depends only on surface orientation,we must assume that the surface is continuous and that itsfirst partial derivatives exist.Most formulations implicitly also require that thefirst partial derivatives be continuous,and some even require that second partial derivatives exist. The existence and continuity of derivatives lends a certain“smoothness”to the surface and allows us to construct local tangent planes.We can then talk about the local surface orientation in terms of the orientation of these tangent planes.There are several commonly used ways of specifying the orientation of a planar surface patch,including:•Unit surface normalˆn[Horn&Brooks86];•Point on the Gaussian sphere[Horn84];•Surface gradient(p,q)[Horn77];•Stereographic coordinates(f,g)[Ikeuchi&Horn81];7The f-number is the ratio of the principal distance to the diameter of the aper-ture,that is,f/d.2.Review of Problem Formulation7•Dip and strike(as defined in geology)8;•Luminance longitude and latitude(as defined in astrogeology)9;•Incident and emittance angles(i and e)10;For our purposes here,the components of the surface gradientp=∂z∂xand q=∂z∂y,(5)will be most directly useful for specifying surface orientation.We can convert between different representations easily.For exam-ple,suppose that we are to determine the unit surface normal given the gradient components.We know that if we move a small distanceδx in x, then the change in height isδz=pδx(since p is the slope of the surface in the x direction).Thus(1,0,p)T is a tangent to the surface.If we move a small distanceδy in y,then the change in height isδz=qδy(since q is the slope of the surface in the y direction).Thus(0,1,q)T is also a tangent to the surface.The normal is perpendicular to all tangents,thus parallel to the cross-product of these particular tangents,that is parallel to(−p,−q,1)T.Hence a unit normal can be written in the formˆn=11+p2+q2(−p,−q,1)T.(6)Note that this assumes that the z-component of the surface normal is pos-itive.This is not a problem since we can only see surface elements whose normal vectors point withinπ/2of the direction toward the viewer—other surface elements are turned away from the viewer.We can use the same notation to specify the direction to a collimated light source or a small portion of an extended source.We simply give the orientation of a surface element that lies perpendicular to the incident8Dip is the angle between a given surface and the horizontal plane,while strike is the direction of the intersection of the surface and the horizontal plane.The line of intersection is perpendicular to the direction of steepest descent.9Luminance longitude and latitude are the longitude and latitude of a point ona sphere with the given orientation,measured in a spherical coordinate systemwith the poles at right angles to both the direction toward the source and the direction toward the viewer.10Incident and emittance angles are meaningful quantities only when there is a single source;and even then there is a two-way ambiguity in surface orienta-tion unless additional information is provided.The same applies to luminance longitude and latitude.8Height and Gradient from Shading light rays.So we can write11ˆs=11+p2s+q2s(−p s,−q s,1)T,(7)for some p s and q s.2.3Reflectance MapWe can show the dependence of scene radiance on surface orientation in the form of a reflectance map R(p,q).The reflectance map can be depicted graphically in gradient space12as a series of nested contours of constant brightness[Horn77,86].The reflectance map may be determined experimentally by mount-ing a sample of the surface on a goniometer stage and measuring its brightness under the given illuminating conditions for various orienta-tions.Alternatively,one may use the image of a calibration object(such as a sphere)for which surface orientation is easily calculated at every point.Finally,a reflectance map may be derived from a phenomenolog-ical model,such as that of a Lambertian surface.In this case one can integrate the product of the bidirectional reflectance distribution function (BRDF)and the given distribution of source brightness as a function of incident angle[Horn&Sjoberg79].An ideal Lambertian surface illuminated by a single point source pro-vides a convenient example of a reflectance map13.Here the scene radi-ance is given by R(p,q)=(E0/π)cos i,where i is the incident angle(the angle between the surface normal and the direction toward the source), while E0is the irradiance from the source on a surface oriented perpendic-ular to the incident rays.(The above formula only applies when i≤π/2; the scene radiance is,of course,zero for i>π/2.)Now cos i=ˆn·ˆs,soR(p,q)=E0π1+p s p+q s q1+p2+q21+p2s+q2s,(8)as long as the numerator is positive,otherwise R(p,q)=0.11There is a small problem,however,with this method for specifying the direction toward the light source:A source may be“behind”the scene,with the direction to the source more thanπ/2away from the direction toward the viewer.In this case the z-component of the vector pointing toward the light source is negative. 12The coordinates of gradient space are p and q,the slopes of the surface in the x and y direction respectively.13Note that shape-from-shading methods are most definitely not restricted to Lambertian surfaces.Such special surfaces merely provide a convenient peda-gogical device for illustrating basic concepts.2.Review of Problem Formulation92.4Image Irradiance EquationWe are now ready to write down the image irradiance equation E(x,y)=βR p(x,y),q(x,y) ,(9)where E(x,y)is the irradiance at the point (x,y)in the image,while R(p,q)is the radiance at the corresponding point in the scene,at which p =p(x,y)and q =q(x,y).The proportionality factor βdepends on the f -number of the imaging system (and may include a scaling factor that depends on the units in which the instrument measures brightness).It is customary to rescale image irradiance so that this proportionality factor may be dropped.If the reflectance map has a unique global extremum,for example,then the image can be normalized in this fashion,provided that a point can be located that has the corresponding surface orientation 14.Scene radiance also depends on the irradiance of the scene and a re-flectance factor (loosely called albedo here).These factors of proportion-ality can be combined into one that can be taken care of by normaliza-tion of image brightness.Then only the geometric dependence of image brightness on surface orientation remains in R(p,q),and we can write the image irradiance equation in the simple form E(x,y)=R p(x,y),q(x,y) (10)orE(x,y)=R z x (x,y),z y (x,y) ,(11)where p =z x and q =z y are the first partial derivatives of z with respect to x and y .This is a first-order partial differential equation;one that is typically nonlinear,because the reflectance map in most cases depends nonlinearly on the gradient.2.5Reflectance Map Linear in GradientViewed from a sufficiently great distance,the material in the maria of the moon has the interesting property that its brightness depends only on luminance longitude,being independent of luminance latitude [Hapke 63,65].When luminance longitude and latitude are related to the incident and emittance angles,it is found that longitude is a function of (cos i/cos e).From the above we see that cos i =ˆn ·ˆs ,while cos e =ˆn ·ˆv ,where ˆv =14If there is a unique maximum in reflected brightness,it is convenient to rescale the measurements so that this extremum corresponds to E =1.The same applies when there is a unique minimum,as is the case for the scanning electron microscope (SEM).10Height and Gradient from Shading (0,0,1)T is a unit vector in the direction toward the viewer.Consequently,cos i cos e =ˆn·ˆsˆn·ˆv=11+p2s+q2s(1+p s p+q s q).(12)Thus(cos i/cos e)depends linearly on the gradient components p and q, and we can writeR(p,q)=f(c p+s q),(13) for some function f and some coefficients c and s.Both Lommel-Seeliger’s and Hapke’s functionsfit this mold[Minnaert61][Hapke63,65].(For a few other papers on the reflecting properties of surfaces,see[Hapke81, 84][Hapke&Wells81]and the bibliography in[Horn&Brooks89].)We can,without loss of generality15,arrange for c2+s2=1.If the function f is continuous and monotonic16,we canfind an in-versec p+s q=f−1E(x,y).(14)The slope in the image direction(c,s)ism=c p+s q√c2+s2=1√c2+s2f−1E(x,y).(15)We can integrate17out this slope along the linex(ξ)=x0+cξand y(ξ)=y0+sξ,(16) to obtainz(ξ)=z0+1√c2+s2ξf−1Ex(η),y(η)dη.(17)An extension of the above approach allows one to take into account per-spective projection as well asfinite distance to the light source[Rind-fleisch66].Two changes need to be made;one is that the reflectance map now is no longer independent of image position(since the directions to the viewer and the source vary significantly);and the other that the integral is for the logarithm of the radial distance from the center of projection, as opposed to distance measured parallel to the optical axis.The above was thefirst shape-from-shading or photoclinometric prob-lem ever solved in other than a heuristic fashion.The original formulation was considerably more complex than described above,as the result of the15We see that c:s=p s:q s,so that the direction specified in the image by(c,s) is the direction“toward the source,”that is,the projection into the image plane of the vectorˆs toward the light source.16If the function f is not monotonic,there will be more than one solution for certain brightness values.In this case one may need to introduce assumptions about continuity of the derivatives in order to decide which solution to choose. 17The integration is,of course,carried out numerically,since the integrand is derived from image measurements and not represented as an analytic function.2.Review of Problem Formulation 11use of full perspective projection,the lack of the notion of anything like the reflectance map,and the use of an object-centered coordinate system[Rindfleisch 66].Note that we obtain profiles of the surface by integrating along pre-determined straight lines in the image.Each profile has its own unknown constant of integration,so there is a great deal of ambiguity in the recov-ery of surface shape.In fact,if z(x,y)is a solution,so isz(x,y)=z(x,y)+g(s x −c y)(18)for an arbitrary function g !This is true becausez x =z x +s g (s x −c y)and z y =z y −c g (s x −c y),(19)so c p +s q =c p +s q,(20)where p =z x and q =z y .It follows that R(p,q)=R(p,q).This ambi-guity can be removed if an initial curve is given from which the profiles can be started.Such an initial curve is typically not available in practice.Ambiguity is not restricted to the special case of a reflectance map that is linear in the gradient:Without additional constraint shape-from-shading problems typically do not have a unique solution.2.6Low Gradient Terrain and Oblique IlluminationIf we are looking at a surface where the gradient (p,q)is small,we can approximate the reflectance map using series expansionR(p,q)≈R(0,0)+p R p (0,0)+q R q (0,0).(21)This approach does not work when the reflectance map is rotationally symmetric,since the first-order terms then drop out 18.If the illumination is oblique,however,we can apply the method in the previous section to get a first estimate of the surface.Letting c =R p (0,0),s =R q (0,0)and f −1 E(x,y) =E(x,y)−R(0,0),(22)we find thatz(ξ)=z 0+1R 2p (0,0)+R 2q (0,0) ξ0 E x(η),y(η) −R(0,0)dη.(23)(For a related frequency domain approach see [Pentland 88].)One might imagine that the above would provide a good way to get initial conditions for an iterative shape from shading method.Unfortu-nately,this is not very helpful,because of the remaining ambiguity in the 18The reflectance map is rotationally symmetric,for example,when the source is where the viewer is,or when an extended source is symmetrically distributed about the direction toward the viewer.12Height and Gradient from Shadingdirection at right angles to that of profile integration.Iterative methods already rapidly get adequate variations in height along“down-sun pro-files,”but then struggle for a long time to try to get these profiles tied together in the direction at right angles.The above also suggests that errors in gradients of a computed so-lution are likely to be small in the direction towards or away“from the source”and large in the direction at right angles.It should also be clear that it is relatively easy tofind solutions for slowly undulating surfaces (where p and q remain small)with oblique illumination(as in[Kirk87]).It is harder to deal with cases where the surface gradient varies widely,and with cases where the source is near the viewer(see also the discussion in section7.3).3.Brief Review of PhotoclinometryPhotoclinometry is the recovery of surface slopes from images[Wilhelms64] [Rindfleisch66][Lambiotte&Taylor67][Watson68][Lucchitta&Gam-bell70][Tyler,Simpson&Moore71][Rowan,McCauley&Holm71][Bonner &Small73][Wildey75][Squyres81][Howard,Blasius&Cutt82].Many pa-pers and abstracts relating to this subject appear in places that may seem inaccessible to someone working in machine vision[Davis,Soderblom, &Eliason82][Passey&Shoemaker82][Davis&McEwen84][Davis& Soderblom83,84][Malin&Danielson84][Wilson et al.84][McEwen85] [Wilson et al.85](For additional references see Shape from Shading[Horn &Brooks89]).Superficially,photoclinometry may appear to be just an-other name for shape from shading.Two different groups of researchers independently tackled the problem of recovering surface shape from spa-tial brightness variations in single images.Astrogeologists and workers in machine vision became aware of each other’s interests only a few years ago.The underlying goals of the two groups are related,but there are some differences in approach that may be worthy of a brief discussion.3.1Photoclinometry versus Shape from Shading•First,photoclinometry has focused mostly on profile methods(pho-toclinometrists now refer to existing shape-from-shading methods as area-based photoclinometry,as opposed to profile-based).This came about in large part because several of the surfaces of interest to the astrogeologist have reflecting properties that allow numeri-cal integration along predetermined lines in the image,as discussed3.Brief Review of Photoclinometry13above in section2.5[Rindfleisch66].Later,a similar profile integra-tion approach was applied to other kinds of surfaces,by using strong assumptions about local surface geometry instead.The assumption that the surface is locally cylindrical leads to such a profile integra-tion scheme[Wildey86],for example.More commonly,however,it has been assumed that the cross-track slope is zero,in a suitable object-centered coordinate system[Squyres81].This may be reason-able when one is considering a cross-section of a linearly extended feature,like a ridge,a graben,or a central section of a rotationally symmetric feature like a crater.•The introduction of constraints that are easiest to express in an object-centered coordinate system leads away from use of a camera-centered coordinate system and to complex coordinate transformations that tend to obscure the underlying problem.A classic paper on photo-clinometry[Rindfleisch66]is difficult to read for this reason,and asa result had little impact on thefield.On the other hand,it must beacknowledged that this paper dealt properly with perspective projec-tion,which is important when thefield of view is large.In all but the earliest work on shape from shading[Horn70,75],the assump-tion is made that the projection is approximately orthographic.This simplifies the equations and allows introduction of the reflectance map.•The inherent ambiguity of the problem does not stand out as ob-viously when one works with profiles,as it does when one tries to fully reconstruct surfaces.This is perhaps why workers on shape from shading have been more concerned with ambiguity,and why they have emphasized the importance of singular points and occlud-ing boundaries[Bruss82][Deift&Sylvester81][Brooks83][Blake, Zisserman&Knowles85][Saxberg88].•The recovery of shape is more complex than the computation of a set of profiles.Consequently much of the work in shape from shading has been restricted to simple shapes.At the same time,there has been extensive testing of shape from shading algorithms on synthetic data.This is something that is important for work on shape from shading, but makes little sense for the study of simple profile methods,except to test for errors in the procedures used for inverting the photometric function.•Shape-from-shading methods easily deal with arbitrary collections of collimated light sources and extended sources,since these can be ac-commodated in the reflectance map by integrating the BRDF and the。
丛生蛋白在肾远曲小管的表达及其与肾结石关系的初步研究
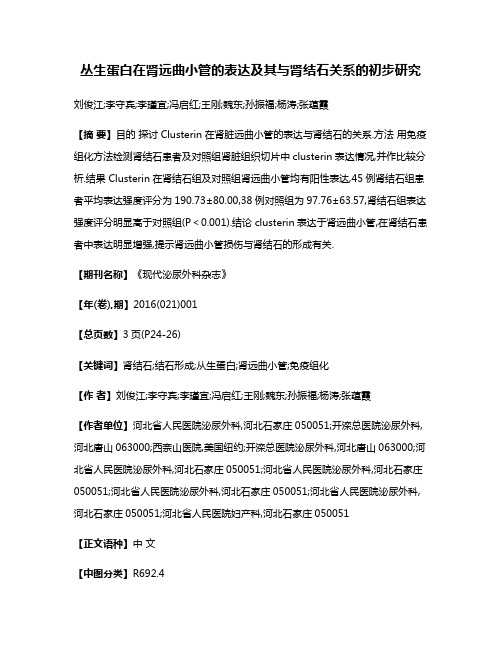
丛生蛋白在肾远曲小管的表达及其与肾结石关系的初步研究刘俊江;李守宾;李瑾宜;冯启红;王刚;魏东;孙振福;杨涛;张蕴霞【摘要】目的探讨Clusterin在肾脏远曲小管的表达与肾结石的关系.方法用免疫组化方法检测肾结石患者及对照组肾脏组织切片中clusterin表达情况,并作比较分析.结果 Clusterin在肾结石组及对照组肾远曲小管均有阳性表达,45例肾结石组患者平均表达强度评分为190.73±80.00,38例对照组为97.76±63.57,肾结石组表达强度评分明显高于对照组(P<0.001).结论 clusterin表达于肾远曲小管,在肾结石患者中表达明显增强,提示肾远曲小管损伤与肾结石的形成有关.【期刊名称】《现代泌尿外科杂志》【年(卷),期】2016(021)001【总页数】3页(P24-26)【关键词】肾结石;结石形成;从生蛋白;肾远曲小管;免疫组化【作者】刘俊江;李守宾;李瑾宜;冯启红;王刚;魏东;孙振福;杨涛;张蕴霞【作者单位】河北省人民医院泌尿外科,河北石家庄050051;开滦总医院泌尿外科,河北唐山063000;西奈山医院,美国纽约;开滦总医院泌尿外科,河北唐山063000;河北省人民医院泌尿外科,河北石家庄050051;河北省人民医院泌尿外科,河北石家庄050051;河北省人民医院泌尿外科,河北石家庄050051;河北省人民医院泌尿外科,河北石家庄050051;河北省人民医院妇产科,河北石家庄050051【正文语种】中文【中图分类】R692.4·临床研究·肾结石是泌尿外科最常见的疾病之一,但是泌尿系结石的发生机制仍不十分明确,关于肾结石形成起源部位也存在争议。
丛生蛋白(Clusterin)是一种广泛存在于人体组织和体液中的凋亡相关蛋白,参与多种生理过程,在很多肾脏损害模型中表达明显升高,且早于肌酐的变化[1]。
肾结石的发生也伴随着肾小管上皮损伤等病理过程,Clusterin是否参与肾结石的发生过程,目前尚无相关研究,为了探讨Clusterin与肾结石发生的关系,我们检测了肾结石患者和非泌尿系结石患者肾组织中Clusterin的表达,并做比较分析。
科谱类的小作文100字左右
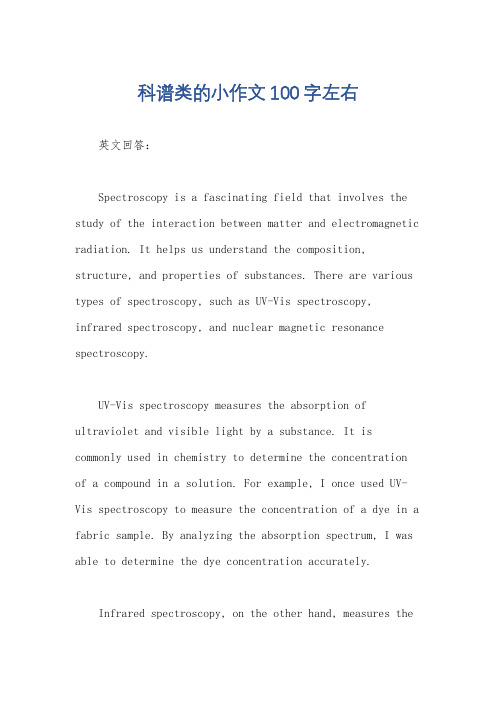
科谱类的小作文100字左右英文回答:Spectroscopy is a fascinating field that involves the study of the interaction between matter and electromagnetic radiation. It helps us understand the composition, structure, and properties of substances. There are various types of spectroscopy, such as UV-Vis spectroscopy,infrared spectroscopy, and nuclear magnetic resonance spectroscopy.UV-Vis spectroscopy measures the absorption of ultraviolet and visible light by a substance. It is commonly used in chemistry to determine the concentration of a compound in a solution. For example, I once used UV-Vis spectroscopy to measure the concentration of a dye in a fabric sample. By analyzing the absorption spectrum, I was able to determine the dye concentration accurately.Infrared spectroscopy, on the other hand, measures theabsorption of infrared light by a substance. It provides information about the functional groups present in a molecule. I remember using infrared spectroscopy toidentify the type of alcohol in a solution. The characteristic peaks in the infrared spectrum helped me determine whether it was a primary, secondary, or tertiary alcohol.Nuclear magnetic resonance (NMR) spectroscopy is a powerful tool for determining the structure of organic molecules. It utilizes the magnetic properties of certain atomic nuclei to provide detailed information about the connectivity and environment of atoms in a molecule. For instance, I used NMR spectroscopy to determine thestructure of an unknown compound. By analyzing the chemical shifts and coupling patterns in the NMR spectrum, I was able to identify the different functional groups present in the molecule.Overall, spectroscopy plays a crucial role in various scientific fields, including chemistry, biochemistry, and materials science. It allows us to analyze and understandthe properties of substances in a non-destructive manner, making it an indispensable tool in research and industry.中文回答:科谱学是一门迷人的领域,涉及物质与电磁辐射之间的相互作用的研究。
A Tutorial on Spectral Clustering
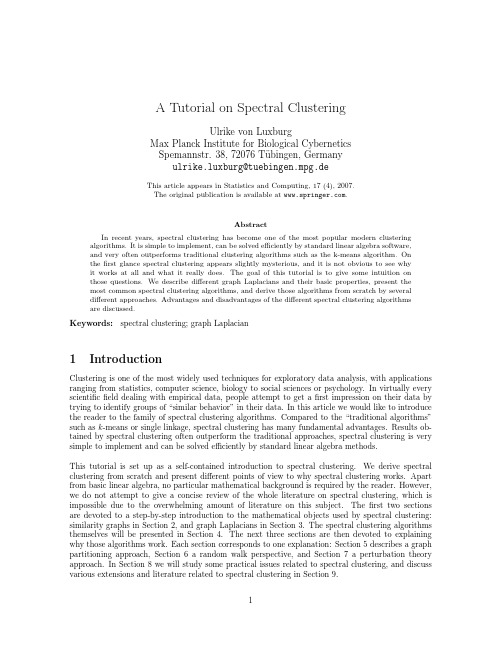
Ulrike von Luxburg Max Planck Institute for Biological Cybernetics Spemannstr. 38, 72076 T¨ ubingen, Germany ulrike.luxburg@tuebingen.mpg.de
2
Similarity graphs
Given a set of data points x1 , . . . xn and some notion of similarity sij ≥ 0 between all pairs of data points xi and xj , the intuitive goal of clustering is to divide the data points into several groups such that points in the same group are similar and points in different groups are dissimilar to each other. If we do not have more information than similarities between data points, a nice way of representing the data is in form of the similarity graph G = (V, E ). Each vertex vi in this graph represents a data point xi . Two vertices are connected if the similarity sij between the corresponding data points xi and xj is positive or larger than a certain threshold, and the edge is weighted by sij . The problem of clustering can now be reformulated using the similarity graph: we want to find a partition of the graph such that the edges between different groups have very low weights (which means that points in different clusters are dissimilar from each other) and the edges within a group have high weights (which means that points within the same cluster are similar to each other). To be able to formalize this intuition we first want to introduce some basic graph notation and briefly discuss the kind of graphs we are going to study.
参考文献——精选推荐
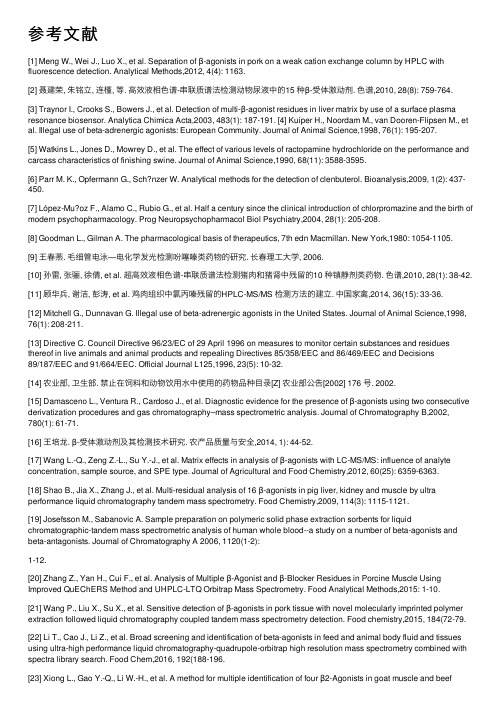
参考⽂献[1] Meng W., Wei J., Luo X., et al. Separation of β-agonists in pork on a weak cation exchange column by HPLC with fluorescence detection. Analytical Methods,2012, 4(4): 1163.[2] 聂建荣, 朱铭⽴, 连槿, 等. ⾼效液相⾊谱-串联质谱法检测动物尿液中的15 种β-受体激动剂. ⾊谱,2010, 28(8): 759-764.[3] Traynor I., Crooks S., Bowers J., et al. Detection of multi-β-agonist residues in liver matrix by use of a surface plasma resonance biosensor. Analytica Chimica Acta,2003, 483(1): 187-191. [4] Kuiper H., Noordam M., van Dooren-Flipsen M., et al. Illegal use of beta-adrenergic agonists: European Community. Journal of Animal Science,1998, 76(1): 195-207.[5] Watkins L., Jones D., Mowrey D., et al. The effect of various levels of ractopamine hydrochloride on the performance and carcass characteristics of finishing swine. Journal of Animal Science,1990, 68(11): 3588-3595.[6] Parr M. K., Opfermann G., Sch?nzer W. Analytical methods for the detection of clenbuterol. Bioanalysis,2009, 1(2): 437-450.[7] López-Mu?oz F., Alamo C., Rubio G., et al. Half a century since the clinical introduction of chlorpromazine and the birth of modern psychopharmacology. Prog Neuropsychopharmacol Biol Psychiatry,2004, 28(1): 205-208.[8] Goodman L., Gilman A. The pharmacological basis of therapeutics, 7th edn Macmillan. New York,1980: 1054-1105.[9] 王春燕. ⽑细管电泳—电化学发光检测吩噻嗪类药物的研究. 长春理⼯⼤学, 2006.[10] 孙雷, 张骊, 徐倩, et al. 超⾼效液相⾊谱-串联质谱法检测猪⾁和猪肾中残留的10 种镇静剂类药物. ⾊谱,2010, 28(1): 38-42.[11] 顾华兵, 谢洁, 彭涛, et al. 鸡⾁组织中氯丙嗪残留的HPLC-MS/MS 检测⽅法的建⽴. 中国家禽,2014, 36(15): 33-36.[12] Mitchell G., Dunnavan G. Illegal use of beta-adrenergic agonists in the United States. Journal of Animal Science,1998, 76(1): 208-211.[13] Directive C. Council Directive 96/23/EC of 29 April 1996 on measures to monitor certain substances and residues thereof in live animals and animal products and repealing Directives 85/358/EEC and 86/469/EEC and Decisions89/187/EEC and 91/664/EEC. Official Journal L125,1996, 23(5): 10-32.[14] 农业部, 卫⽣部. 禁⽌在饲料和动物饮⽤⽔中使⽤的药物品种⽬录[Z] 农业部公告[2002] 176 号. 2002.[15] Damasceno L., Ventura R., Cardoso J., et al. Diagnostic evidence for the presence of β-agonists using two consecutive derivatization procedures and gas chromatography–mass spectrometric analysis. Journal of Chromatography B,2002,780(1): 61-71.[16] 王培龙. β-受体激动剂及其检测技术研究. 农产品质量与安全,2014, 1): 44-52.[17] Wang L.-Q., Zeng Z.-L., Su Y.-J., et al. Matrix effects in analysis of β-agonists with LC-MS/MS: influence of analyte concentration, sample source, and SPE type. Journal of Agricultural and Food Chemistry,2012, 60(25): 6359-6363.[18] Shao B., Jia X., Zhang J., et al. Multi-residual analysis of 16 β-agonists in pig liver, kidney and muscle by ultra performance liquid chromatography tandem mass spectrometry. Food Chemistry,2009, 114(3): 1115-1121.[19] Josefsson M., Sabanovic A. Sample preparation on polymeric solid phase extraction sorbents for liquid chromatographic-tandem mass spectrometric analysis of human whole blood--a study on a number of beta-agonists and beta-antagonists. Journal of Chromatography A 2006, 1120(1-2):1-12.[20] Zhang Z., Yan H., Cui F., et al. Analysis of Multiple β-Agonist and β-Blocker Residues in Porcine Muscle Using Improved QuEChERS Method and UHPLC-LTQ Orbitrap Mass Spectrometry. Food Analytical Methods,2015: 1-10. [21] Wang P., Liu X., Su X., et al. Sensitive detection of β-agonists in pork tissue with novel molecularly imprinted polymer extraction followed liquid chromatography coupled tandem mass spectrometry detection. Food chemistry,2015, 184(72-79.[22] Li T., Cao J., Li Z., et al. Broad screening and identification of beta-agonists in feed and animal body fluid and tissues using ultra-high performance liquid chromatography-quadrupole-orbitrap high resolution mass spectrometry combined with spectra library search. Food Chem,2016, 192(188-196.[23] Xiong L., Gao Y.-Q., Li W.-H., et al. A method for multiple identification of four β2-Agonists in goat muscle and beefmuscle meats using LC-MS/MS based on deproteinization by adjusting pH and SPE for sample cleanup. Food Science and Biotechnology,2015, 24(5): 1629-1635.[24] Zhang Y., Zhang Z., Sun Y., et al. Development of an Analytical Method for the Determination of β2-Agonist Residues in Animal Tissues by High-Performance Liquid Chromatography with On-line Electrogenerated [Cu (HIO6) 2] 5--Luminol Chemiluminescence Detection. Journal of Agricultural and Food chemistry,2007, 55(13): 4949-4956.[25] Liu W., Zhang L., Wei Z., et al. Analysis of beta-agonists and beta-blockers in urine using hollow fibre-protected liquid-phase microextraction with in situ derivatization followed by gas chromatography/mass spectrometry. Journal of Chromatography A 2009, 1216(28): 5340-5346. [26] Caban M., Mioduszewska K., Stepnowski P., et al. Dimethyl(3,3,3-trifluoropropyl)silyldiethylamine--a new silylating agent for the derivatization of beta-blockers and beta-agonists in environmental samples. Analytica Chimica Acta,2013, 782(75-88.[27] Caban M., Stepnowski P., Kwiatkowski M., et al. Comparison of the Usefulness of SPE Cartridges for the Determination of β-Blockers and β-Agonists (Basic Drugs) in Environmental Aqueous Samples. Journal of Chemistry,2015, 2015([28] Zhang Y., Wang F., Fang L., et al. Rapid determination of ractopamine residues in edible animal products by enzyme-linked immunosorbent assay: development and investigation of matrix effects. J Biomed Biotechnol,2009, 2009(579175.[29] Roda A., Manetta A. C., Piazza F., et al. A rapid and sensitive 384-microtiter wells format chemiluminescent enzyme immunoassay for clenbuterol. Talanta,2000, 52(2): 311-318.[30] Bacigalupo M., Meroni G., Secundo F., et al. Antibodies conjugated with new highly luminescent Eu 3+ and Tb 3+ chelates as markers for time resolved immunoassays. Application to simultaneous determination of clenbuterol and free cortisol in horse urine. Talanta,2009, 80(2): 954-958.[31] He Y., Li X., Tong P., et al. An online field-amplification sample stacking method for the determination of β 2-agonists in human urine by CE-ESI/MS. Talanta,2013, 104(97-102.[32] Li Y., Niu W., Lu J. Sensitive determination of phenothiazines in pharmaceutical preparation and biological fluid by flow injection chemiluminescence method using luminol–KMnO 4 system. Talanta,2007, 71(3): 1124-1129.[33] Saar E., Beyer J., Gerostamoulos D., et al. The analysis of antipsychotic drugs in humanmatrices using LC‐MS (/MS). Drug testing and analysis,2012, 4(6): 376-394.[34] Mallet E., Bounoure F., Skiba M., et al. Pharmacokinetic study of metopimazine by oral route in children. Pharmacol Res Perspect,2015, 3(3): e00130.[35] Thakkar R., Saravaia H., Shah A. Determination of Antipsychotic Drugs Known for Narcotic Action by Ultra Performance Liquid Chromatography. Analytical Chemistry Letters,2015, 5(1): 1-11.[36] Kumazawa T., Hasegawa C., Uchigasaki S., et al. Quantitative determination of phenothiazine derivatives in human plasma using monolithic silica solid-phase extraction tips and gas chromatography–mass spectrometry. Journal of Chromatography A,2011, 1218(18): 2521-2527.[37] Flieger J., Swieboda R. Application of chaotropic effect in reversed-phase liquid chromatography of structurally related phenothiazine and thioxanthene derivatives. J Chromatogr A,2008, 1192(2): 218-224.[38] Tu Y. Y., Hsieh M. M., Chang S. Y. Sensitive detection of piperazinyl phenothiazine drugs by field‐amplified sample stacking in capillary electrophoresis with dispersive liquid–liquid microextraction. Electrophoresis,2015, 36(21-22): 2828-2836.[39] Geiser L., Veuthey J. L. Nonaqueous capillary electrophoresis in pharmaceutical analysis. Electrophoresis,2007, 28(1‐2): 45-57.[40] Lara F. J., García‐Campa?a A. M., Gámiz‐Gracia L., et al. Determination of phenothiazines in pharmaceutical formulations and human urine using capillary electrophoresis with chemiluminescence detection. Electrophoresis,2006,27(12): 2348-2359.[41] Lee H. B., Sarafin K., Peart T. E. Determination of beta-blockers and beta2-agonists in sewage by solid-phase extraction and liquid chromatography-tandem mass spectrometry. J Chromatogr A,2007, 1148(2): 158-167.[42] Meng W., Wei J., Luo X., et al. Separation of β-agonists in pork on a weak cation exchange column by HPLC with fluorescence detection. Analytical Methods,2012, 4(4): 1163-1167. [43] Yang F., Liu Z., Lin Y., et al. Development an UHPLC-MS/MS Method for Detection of β-Agonist Residues in Milk. Food Analytical Methods,2011, 5(1): 138-147.[44] Quintana M., Blanco M., Lacal J., et al. Analysis of promazines in bovine livers by high performance liquid chromatography with ultraviolet and fluorimetric detection. Talanta,2003, 59(2): 417-422.[45] Tanaka E., Nakamura T., Terada M., et al. Simple and simultaneous determination for 12 phenothiazines in human serum by reversed-phase high-performance liquid chromatography. J Chromatogr B Analyt Technol Biomed Life Sci,2007, 854(1-2): 116-120.[46] Kumazawa T., Hasegawa C., Uchigasaki S., et al. Quantitative determination of phenothiazine derivatives in human plasma using monolithic silica solid-phase extraction tips and gas chromatography-mass spectrometry. J ChromatogrA,2011, 1218(18): 2521-2527.[47] Qian J. X., Chen Z. G. A novel electromagnetic induction detector with a coaxial coil for capillary electrophoresis. Chinese Chemical Letters,2012, 23(2): 201-204.[48] Baciu T., Botello I., Borrull F., et al. Capillary electrophoresis and related techniques in the determination of drugs of abuse and their metabolites. TrAC Trends in Analytical Chemistry,2015, 74(89-108.[49] Sirichai S., Khanatharana P. Rapid analysis of clenbuterol, salbutamol, procaterol, and fenoterol in pharmaceuticals and human urine by capillary electrophoresis. Talanta,2008, 76(5):1194-1198.[50] Toussaint B., Palmer M., Chiap P., et al. On‐line coupling of partial filling‐capillary zone electrophoresis with mass spectrometry for the separation of clenbuterol enantiomers. Electrophoresis,2001, 22(7): 1363-1372.[51] Redman E. A., Mellors J. S., Starkey J. A., et al. Characterization of Intact Antibody Drug Conjugate Variants using Microfluidic CE-MS. Analytical chemistry,2016.[52] Ji X., He Z., Ai X., et al. Determination of clenbuterol by capillary electrophoresis immunoassay with chemiluminescence detection. Talanta,2006, 70(2): 353-357.[53] Li L., Du H., Yu H., et al. Application of ionic liquid as additive in determination of three beta-agonists by capillary electrophoresis with amperometric detection. Electrophoresis,2013, 34(2): 277-283.[54] 张维冰. ⽑细管电⾊谱理论基础. 北京:科学出版社,2006.[55] Anurukvorakun O., Suntornsuk W., Suntornsuk L. Factorial design applied to a non-aqueous capillary electrophoresis method for the separation of beta-agonists. J Chromatogr A,2006, 1134(1-2): 326-332.[56] Shi Y., Huang Y., Duan J., et al. Field-amplified on-line sample stacking for separation and determination of cimaterol, clenbuterol and salbutamol using capillary electrophoresis. J Chromatogr A,2006, 1125(1): 124-128.[57] Chevolleau S., Tulliez J. Optimization of the separation of β-agonists by capillary electrophoresis on untreated and C 18 bonded silica capillaries. Journal of Chromatography A,1995, 715(2): 345-354.[58] Wang W., Zhang Y., Wang J., et al. Determination of beta-agonists in pig feed, pig urine and pig liver using capillary electrophoresis with electrochemical detection. Meat Sci,2010, 85(2): 302-305.[59] Lin C. E., Liao W. S., Chen K. H., et al. Influence of pH on electrophoretic behavior of phenothiazines and determination of pKa values by capillary zone electrophoresis. Electrophoresis,2003, 24(18): 3154-3159.[60] Muijselaar P., Claessens H., Cramers C. Determination of structurally related phenothiazines by capillary zone electrophoresis and micellar electrokinetic chromatography. Journal of Chromatography A,1996, 735(1): 395-402.[61] Wang R., Lu X., Xin H., et al. Separation of phenothiazines in aqueous and non-aqueous capillary electrophoresis. Chromatographia,2000, 51(1-2): 29-36.[62] Chen K.-H., Lin C.-E., Liao W.-S., et al. Separation and migration behavior of structurally related phenothiazines in cyclodextrin-modified capillary zone electrophoresis. Journal of Chromatography A,2002, 979(1): 399-408.[63] Lara F. J., Garcia-Campana A. M., Ales-Barrero F., et al. Development and validation of a capillary electrophoresis method for the determination of phenothiazines in human urine in the low nanogram per milliliter concentration range using field-amplified sample injection. Electrophoresis,2005, 26(12): 2418-2429.[64] Lara F. J., Garcia-Campana A. M., Gamiz-Gracia L., et al. Determination of phenothiazines in pharmaceutical formulations and human urine using capillary electrophoresis with chemiluminescence detection. Electrophoresis,2006,27(12): 2348-2359.[65] Yu P. L., Tu Y. Y., Hsieh M. M. Combination of poly(diallyldimethylammonium chloride) and hydroxypropyl-gamma-cyclodextrin for high-speed enantioseparation of phenothiazines bycapillary electrophoresis. Talanta,2015, 131(330-334.[66] Kakiuchi T. Mutual solubility of hydrophobic ionic liquids and water in liquid-liquid two-phase systems for analytical chemistry. Analytical Sciences,2008, 24(10): 1221-1230.[67] 陈志涛. 基于离⼦液体相互作⽤⽑细管电泳新⽅法. 万⽅数据资源系统, 2011.[68] Liu J.-f., Jiang G.-b., J?nsson J. ?. Application of ionic liquids in analytical chemistry. TrAC Trends in Analytical Chemistry,2005, 24(1): 20-27.[69] YauáLi S. F. Electrophoresis of DNA in ionic liquid coated capillary. Analyst,2003, 128(1): 37-41.[70] Kaljurand M. Ionic liquids as electrolytes for nonaqueous capillary electrophoresis. Electrophoresis,2002, 23(426-430.[71] Xu Y., Gao Y., Li T., et al. Highly Efficient Electrochemiluminescence of Functionalized Tris (2, 2′‐bipyridyl) ruthenium (II) and Selective Concentration Enrichment of Its Coreactants. Advanced Functional Materials,2007, 17(6): 1003-1009.[72] Pandey S. Analytical applications of room-temperature ionic liquids: a review of recent efforts. Anal Chim Acta,2006, 556(1): 38-45.[73] Koel M. Ionic Liquids in Chemical Analysis. Critical Reviews in Analytical Chemistry,2005, 35(3): 177-192.[74] Yanes E. G., Gratz S. R., Baldwin M. J., et al. Capillary electrophoretic application of 1-alkyl-3-methylimidazolium-based ionic liquids. Analytical chemistry,2001, 73(16): 3838-3844.[75] Qi S., Cui S., Chen X., et al. Rapid and sensitive determination of anthraquinones in Chinese herb using 1-butyl-3-methylimidazolium-based ionic liquid with β-cyclodextrin as modifier in capillary zone electrophoresis. Journal of Chromatography A,2004, 1059(1-2): 191-198.[76] Jiang T.-F., Gu Y.-L., Liang B., et al. Dynamically coating the capillary with 1-alkyl-3-methylimidazolium-based ionic liquids for separation of basic proteins by capillary electrophoresis. Analytica Chimica Acta,2003, 479(2): 249-254.[77] Jiang T. F., Wang Y. H., Lv Z. H. Dynamic coating of a capillary with room-temperature ionic liquids for the separation of amino acids and acid drugs by capillary electrophoresis. Journal of Analytical Chemistry,2006, 61(11): 1108-1112.[78] Qi S., Cui S., Cheng Y., et al. Rapid separation and determination of aconitine alkaloids in traditional Chinese herbs by capillary electrophoresis using 1-butyl-3-methylimidazoium-based ionic liquid as running electrolyte. Biomed Chromatogr,2006, 20(3): 294-300.[79] Wu X., Wei W., Su Q., et al. Simultaneous separation of basic and acidic proteins using 1-butyl-3-methylimidazolium-based ion liquid as dynamic coating and background electrolyte in capillary electrophoresis. Electrophoresis,2008, 29(11): 2356-2362.[80] Guo X. F., Chen H. Y., Zhou X. H., et al. N-methyl-2-pyrrolidonium methyl sulfonate acidic ionic liquid as a new dynamic coating for separation of basic proteins by capillary electrophoresis. Electrophoresis,2013, 34(24): 3287-3292.[81] Mo H., Zhu L., Xu W. Use of 1-alkyl-3-methylimidazolium-based ionic liquids as background electrolytes in capillary electrophoresis for the analysis of inorganic anions. J Sep Sci,2008, 31(13): 2470-2475.[82] Yu L., Qin W., Li S. F. Y. Ionic liquids as additives for separation of benzoic acid and chlorophenoxy acid herbicides by capillary electrophoresis. Analytica Chimica Acta,2005, 547(2): 165-171.[83] Marszall M. P., Markuszewski M. J., Kaliszan R. Separation of nicotinic acid and itsstructural isomers using 1-ethyl-3-methylimidazolium ionic liquid as a buffer additive by capillary electrophoresis. J Pharm Biomed Anal,2006, 41(1): 329-332.[84] Gao Y., Xu Y., Han B., et al. Sensitive determination of verticine and verticinone in Bulbus Fritillariae by ionic liquid assisted capillary electrophoresis-electrochemiluminescence system. Talanta,2009, 80(2): 448-453.[85] Li J., Han H., Wang Q., et al. Polymeric ionic liquid as a dynamic coating additive for separation of basic proteins by capillary electrophoresis. Anal Chim Acta,2010, 674(2): 243-248.[86] Su H. L., Kao W. C., Lin K. W., et al. 1-Butyl-3-methylimidazolium-based ionic liquids and an anionic surfactant: excellentbackground electrolyte modifiers for the analysis of benzodiazepines through capillary electrophoresis. J ChromatogrA,2010, 1217(17): 2973-2979.[87] Huang L., Lin J. M., Yu L., et al. Improved simultaneous enantioseparation of beta-agonists in CE using beta-CD and ionic liquids. Electrophoresis,2009, 30(6): 1030-1036.[88] Laamanen P. L., Busi S., Lahtinen M., et al. A new ionic liquid dimethyldinonylammonium bromide as a flow modifier for the simultaneous determination of eight carboxylates by capillary electrophoresis. J Chromatogr A,2005, 1095(1-2): 164-171.[89] Yue M.-E., Shi Y.-P. Application of 1-alkyl-3-methylimidazolium-based ionic liquids in separation of bioactive flavonoids by capillary zone electrophoresis. Journal of Separation Science,2006, 29(2): 272-276.[90] Liu C.-Y., Ho Y.-W., Pai Y.-F. Preparation and evaluation of an imidazole-coated capillary column for the electrophoretic separation of aromatic acids. Journal of Chromatography A,2000, 897(1): 383-392.[91] Qin W., Li S. F. An ionic liquid coating for determination of sildenafil and UK‐103,320 in human serum by capillary zone electrophoresis‐ion trap mass spectrometry. Electrophoresis,2002, 23(24): 4110-4116.[92] Qin W., Li S. F. Y. Determination of ammonium and metal ions by capillary electrophoresis–potential gradient detection using ionic liquid as background electrolyte and covalent coating reagent. Journal of Chromatography A,2004, 1048(2): 253-256.[93] Borissova M., Vaher M., Koel M., et al. Capillary zone electrophoresis on chemically bonded imidazolium based salts. J Chromatogr A,2007, 1160(1-2): 320-325.[94] Vaher M., Koel M., Kaljurand M. Non-aqueous capillary electrophoresis in acetonitrile using lonic-liquid buffer electrolytes. Chromatographia,2000, 53(1): S302-S306.[95] Vaher M., Koel M., Kaljurand M. Ionic liquids as electrolytes for nonaqueous capillary electrophoresis. Electrophoresis,2002, 23(3): 426.[96] Vaher M., Koel M. Separation of polyphenolic compounds extracted from plant matrices using capillary electrophoresis. Journal of Chromatography A,2003, 990(1-2): 225-230.[97] Francois Y., Varenne A., Juillerat E., et al. Nonaqueous capillary electrophoretic behavior of 2-aryl propionic acids in the presence of an achiral ionic liquid. A chemometric approach. J Chromatogr A,2007, 1138(1-2): 268-275.[98] Lamoree M., Reinhoud N., Tjaden U., et al. On‐capillary isotachophoresis for loadability enhancement in capillary zone electrophoresis/mass spectrometry of β‐agonists. Biological mass spectrometry,1994, 23(6): 339-345.[99] Huang P., Jin X., Chen Y., et al. Use of a mixed-mode packing and voltage tuning for peptide mixture separation in pressurized capillary electrochromatography with an ion trap storage/reflectron time-of-flight mass spectrometer detector. Analytical chemistry,1999, 71(9):1786-1791.[100] Le D. C., Morin C. J., Beljean M., et al. Electrophoretic separations of twelve phenothiazines and N-demethyl derivatives by using capillary zone electrophoresis and micellar electrokinetic chromatography with non ionic surfactant. Journal of Chromatography A,2005, 1063(1-2): 235-240.。
Star-FormingNuclearRingsinSpiralGalaxies
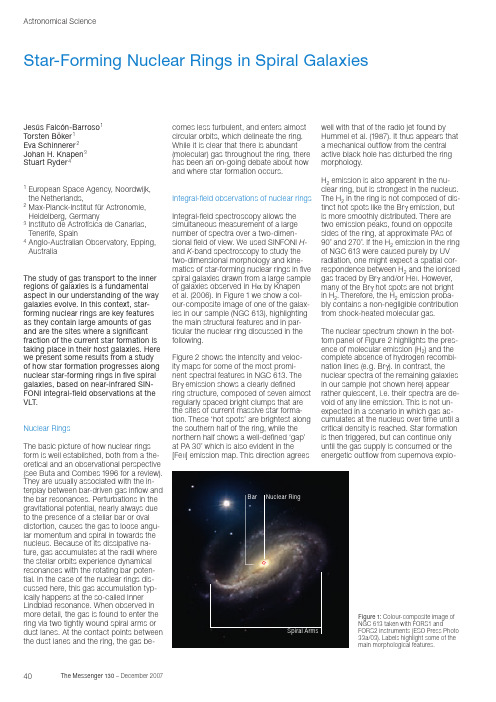
Astronomical ScienceStar-Forming Nuclear Rings in Spiral GalaxiesJesús Falcón-Barroso1Torsten Böker1Eva Schinnerer2Johan H. Knapen3Stuart Ryder41E uropean Space Agency, Noordwijk, the Netherlands,2M ax-Planck-Institut für Astronomie,H eidelberg, Germany3I nstituto de Astrofísica de Canarias, Tenerife, Spain4A nglo-Australian Observatory, Epping, AustraliaThe study of gas transport to the inner regions of galaxies is a fundamentala spect in our understanding of the way galaxies evolve. In this context, star-forming nuclear rings are key features as they contain large amounts of gas and are the sites where a significant fraction of the current star formation is taking place in their host galaxies. Here we present some results from a study of how star formation progresses along nuclear star-forming rings in five spiral galaxies, based on near-infrared SIN-FONI integral-field observations at the VLT.Nuclear RingsThe basic picture of how nuclear rings form is well established, both from a the-oretical and an observational perspective (see Buta and Combes 1996 for a review). They are usually associated with the in-terplay between bar-driven gas inflow and the bar resonances. Perturbations in the gravitational potential, nearly always due to the presence of a stellar bar or oval distortion, causes the gas to loose angu-lar momentum and spiral in towards the nucleus. Because of its dissipative na-ture, gas accumulates at the radii where the stellar orbits experience dynamical resonances with the rotating bar poten-tial. In the case of the nuclear rings dis-cussed here, this gas accumulation typ-ically happens at the so-called Inner Lindblad resonance. When observed in more detail, the gas is found to enter the ring via two tightly wound spiral arms or dust lanes. At the contact points between the dust lanes and the ring, the gas be-comes less turbulent, and enters almostcircular orbits, which delineate the ring.While it is clear that there is abundant(mo l ecular) gas throughout the ring, therehas been an on-going debate about howand where star formation occurs.Integral-field observations of nuclear ringsIntegral-field spectroscopy allows thes imultaneous measurement of a largenumber of spectra over a two-dimen-sional field of view. We used SINFONI H-and K-band spectroscopy to study thetwo-dimensional morphology and kine-matics of star-forming nuclear rings in fivespiral galaxies drawn from a large sampleof galaxies observed in H a by Knapenet al. (2006). In Figure 1 we show a col-our-composite image of one of the galax-ies in our sample (NGC 613), highlightingthe main structural features and in par-ticular the nuclear ring discussed in thefollowing.Figure 2 shows the intensity and veloc -ity maps for some of the most promi-nent spectral features in NGC 613. TheBr g emission shows a clearly definedring structure, composed of seven almostregularly spaced bright clumps that arethe sites of current massive star forma-tion. These ‘hot spots’ are brightest alongthe southern half of the ring, while thenorthern half shows a well-defined ‘gap’at PA 30˚ which is also evident in the[Fe ii] emission map. This direction agreeswell with that of the radio jet found byHummel et al. (1987). It thus appears thata mechanical outflow from the centrala ctive black hole has disturbed the ringmorphology.H2 emission is also apparent in the nu-clear ring, but is strongest in the nucleus.The H2 in the ring is not composed of dis-tinct hot spots like the Br g emission, butis more smoothly distributed. There aretwo emission peaks, found on oppositesides of the ring, at approximate PAs of90˚ and 270˚. If the H2 emission in the ringof NGC 613 were caused purely by UVradiation, one might expect a spatial cor-respondence between H2 and the ionisedgas traced by Br g and/or He i. However,many of the Br g hot spots are not brightin H2. Therefore, the H2 emission proba-bly contains a non-negligible contributionfrom shock-heated molecular gas.The nuclear spectrum shown in the bot-tom panel of Figure 2 highlights the pres-ence of molecular emission (H2) and thecomplete absence of hydrogen recombi-nation lines (e.g. Br g). In contrast, then uclear spectra of the remaining galaxiesin our sample (not shown here) appearrather quiescent, i.e. their spectra are de-void of any line emission. This is not un-expected in a scenario in which gas ac-cumulates at the nucleus over time until acritical density is reached. Star formationis then triggered, but can continue onlyuntil the gas supply is consumed or theenergetic outflow from supernova explo-Figure 1: Colour-composite image ofNGC 613 taken with FORS1 andFORS2 instruments (ESO Press Photo33a/03). Labels highlight some of themain morphological features.Nuclear RingBarSpiral Arms40The Messenger 130 – December 200741The Messenger 130 – December 2007‘quenching’ the star formation. This sce-nario is entirely consistent with observa-tions, at least for late-type spirals: only about 10% of nuclear star clusters cur-rently show emission lines, although most of them harbour a young stellar popula-tion of less than 100 Myr old (Rossa et al. 2006). This can naturally be explained if star formation in galactic nuclei is epi-sodic in nature, with a duty cycle of about 10%.In Figure 2 we also show the stellar and ionised-gas kinematics for NGC 613. The stellar velocity field appears rather regular, despite the intense star forma -tion within the nuclear ring. Because Br g is generally the most prominent emis- sion line in our data, we used it to make an estimate of the ionised gas kinemat -ics. In all galaxies of our sample, the ve-locity gradients within the nuclear rings are smooth and the ionised gas has the same sense of rotation as the stars.Measuring the relative ages of the differ-ent stellar clusters (i.e. hot spots) along the ring is a difficult task. The orbital time-scales in the ring are short (e.g. a few tens of Myr). In order to perform the age dating, it is therefore imperative that one uses tracers that are sensitive to these time-scales. The most widely used diag-nostic for this purpose is the H a emission line. There are several reasons why this line is a popular choice. First, it is an opti-cal emission line that can easily be meas-ured. Second, its equivalent width (EW) decreases almost monotonically with time for an instantaneous burst allowing one to determine the evolutionary stage of a cluster from the EW value alone. The use of this emission line, however, is limited to clusters in the age range be-tween 3 and 10 Myr, and therefore any in-ferred age differences have to be small (few Myr). Below 3 Myr, the EW of the H a is almost constant, and above 10 Myr thevalue is well below typical detection limits (~ 1 Å).An alternative approach is to make use of several spectral lines, whose emission peaks at different cluster ages. In our case, we use the flux of three emission lines that are prominent in the NIR spec-tra of the star-forming regions in ours ample of galaxies: He i , Br g , and [Fe ii ]. Under the assumption that the underly-ing bulge/disc can be considered quies-cent, and that most of the emission is produced in the ring itself, line fluxes are a better probe than the EWs. The reason is that EWs require an accurate knowl-edge of the continuum emission, not only for the hot spot itself, but also for the un-derlying bulge and/or disc, which is very hard to measure. The He i and Br g lines are both produced by photoionisation in the vicinity of hot O- or B-type stars. Given that the ionisation energy for the He i line is higher than that of Br g , it re-quires the presence of hotter and more massive stars, and hence its brightness falls off more rapidly after an instantane-ous burst than that of the Br g line. The time range covered by these two lines is from 0 to 10 Myr. Larger ages can be probed with the [Fe ii ] line (a tracer of fast shocks produced in supernova remnants)whose contribution is almost constant from 3 to 35 Myr, and decreases sharply after that. Using the relative strength of those three lines, we are therefore able to probe ages in the range between 0 and 35 Myr, which represents a good match to the expected travel time of gas and star clusters along the nuclear ring. Star formation along the ringsFigure 3 illustrates two plausible mecha-nisms that describe how star formation could proceed along the circumnuclear rings. In the first scenario, the popcorn model, gas enters the ring and accumu-1.0E -16/5.0E -14–22a r c s e cFe II–202arcsec–22a r c s e c1.0E -16/2.5E -14H 21.0E -16/5.0E -14He I arcsec1.0E -16/5.0E -14Br γarcsecFigure 2: SINFONI near-infrared integral-field spec-troscopy of NGC 613. Top row shows the morphol-ogy in the [Fe ii ], He i and Brackett-g emission; lower row the H 2 emission morphology, the stellar kine-matics (V(star)) and the Brackett-g kinematics (V(Br g )) of the nuclear ring in this galaxy. The black contours delineate the K -band light measured from the SIN-FONI observations while ellipses in the Br g map de-limit the extension of the ring. Bottom panel shows the nuclear spectrum of NGC 613 with the main spectral features highlighted.42The Messenger 130 – December 2007Astronomical Science Falcón-Barroso J. et al., Star-Forming Nuclear Rings in Spiral GalaxiesFigure 3: Two possible scenarios describing how star formation pro g resses along a nuclear ring. Left: the popcorn model, where star formation occurs randomly along the ring. Right: the pearls on a string scenario, where the ages of the stellar cluster de- fine two sequences of increasing age along the ring. (Courtesy of J. Paillet, ESA)lates around it with no preferred location. Once a critical density is reached, the gas becomes unstable to gravitational collapse and star formation is triggered. In this model, individual hot spots col-lapse at random times and locations within the ring, and therefore there is no systematic age sequence. In the figure (left plot) the different starbursts are de-noted by the star symbols and the differ-ent colours indicate different ages of the hot spots. In the second scenario (right), gas enters the ring at the intersection be-tween the bar major axis and the inner Lindblad resonance. Downstream from this location, at the so-called over-den-sity region (ODR), the gas density be-comes sufficiently high to ignite star for-mation in a short-lived burst. A young cluster formed there will continue its orbit around the ring, but star formation will cease as soon as the first supernova ex-plosions expel the gas. A series of star-bursts triggered in the ODR will then pro-duce a sequence of star clusters thatenter the ring like pearls on a string . In this scenario, the star clusters should show a bipolar age gradient along the ring, with the youngest clusters found close to the ODR, and increasingly older cluster ages in the direction of rotation, up to the opposite ODR. This is shown in the figure, where the colour sequence blue-green-red denotes clusters with in-creasing age.In Figure 4 we put these two models to the test by showing the observations for NGC 613. In the left panel we display an F666W HST image of the nuclear regions. The ring is outlined with an ellipse, and two star symbols mark roughly the posi-tions of the ODRs (based on the regions with highest dust extinction in the ring). In the right panel we present a false-col-our image constructed from the SINFONI emission line maps of He i , Br g , and [Fe ii ] assigned to the blue, green, and redc olour channels, respectively. The bot-tom half of the ring shows the trends ex-odrodrpected under the pearls on a string pic-ture: an age sequence (blue-green-red) in the different hot spots. The sequence is less obvious in the top part of the ring due to the strong interaction between the ring and the radio jet. In the full sample, not shown here, three out of five galaxies show some evidence for an age gradient of hot spots along the ring, while the re-maining two galaxies have incompletei nformation and thus are consistent withe ither model. A more detailed account of the results presented here can be found in Böker et al. (2007).ReferencesBöker T. et al. 2007, AJ, in pressButa R. and Combes F. 1996, Fund. Cosmic Physics 17, 95Hummel E. et al. 1987, A&A 172, 51Knapen J. et al. 2006, A&A 448, 489Rossa J. et al. 2006, AJ 132, 1074Figure 4: Evidence in support of the pearls on astring scenario in NGC 613. Left: HST F666W image. Right: He i -Br g -Fe ii (blue-green-red) composite image of NGC 613 nuclear ring. Solid ellipse lines mark the lo c ation of the ring, star symbols the po-sitions of the over-dense regions (ODRs), and arrows the sense of rotation of the stars and gas in the gal-axy.A ‘Popcorn’B‘Pearls on a string’。
Spectroscopy and Spectral Analysis
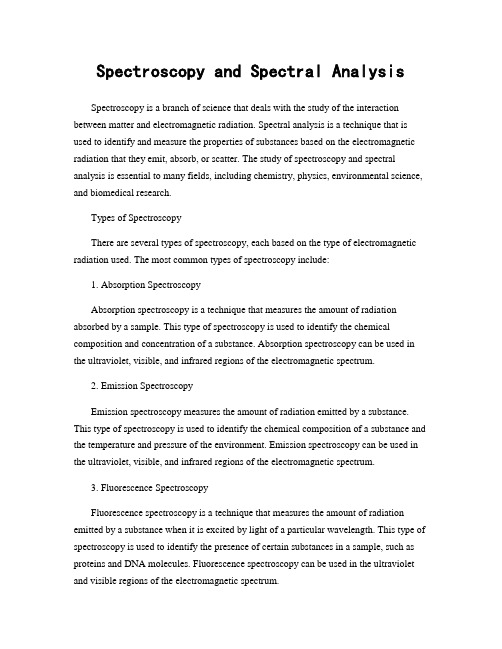
Spectroscopy and Spectral AnalysisSpectroscopy is a branch of science that deals with the study of the interaction between matter and electromagnetic radiation. Spectral analysis is a technique that is used to identify and measure the properties of substances based on the electromagnetic radiation that they emit, absorb, or scatter. The study of spectroscopy and spectral analysis is essential to many fields, including chemistry, physics, environmental science, and biomedical research.Types of SpectroscopyThere are several types of spectroscopy, each based on the type of electromagnetic radiation used. The most common types of spectroscopy include:1. Absorption SpectroscopyAbsorption spectroscopy is a technique that measures the amount of radiation absorbed by a sample. This type of spectroscopy is used to identify the chemical composition and concentration of a substance. Absorption spectroscopy can be used in the ultraviolet, visible, and infrared regions of the electromagnetic spectrum.2. Emission SpectroscopyEmission spectroscopy measures the amount of radiation emitted by a substance. This type of spectroscopy is used to identify the chemical composition of a substance and the temperature and pressure of the environment. Emission spectroscopy can be used in the ultraviolet, visible, and infrared regions of the electromagnetic spectrum.3. Fluorescence SpectroscopyFluorescence spectroscopy is a technique that measures the amount of radiation emitted by a substance when it is excited by light of a particular wavelength. This type of spectroscopy is used to identify the presence of certain substances in a sample, such as proteins and DNA molecules. Fluorescence spectroscopy can be used in the ultraviolet and visible regions of the electromagnetic spectrum.4. Raman SpectroscopyRaman spectroscopy is a technique that measures the scattered radiation produced when a sample is irradiated with a laser beam. This type of spectroscopy is used to identify the chemical composition and structure of a substance. Raman spectroscopy can be used in the visible and near-infrared regions of the electromagnetic spectrum.Applications of Spectroscopy and spectral analysis have a wide range of applications in various fields, including:1. ChemistrySpectroscopy is used extensively in chemistry to identify the chemical composition and properties of substances. Spectroscopy is used to determine the purity of a substance, study chemical reactions, and analyze the structure of molecules.2. PhysicsIn physics, spectroscopy is used to study the properties of materials, such as their electronic and magnetic properties. Spectroscopy is used to study the interactions between atoms and molecules and to investigate the behavior of quantum systems.3. Environmental ScienceSpectroscopy is used in environmental science to study the properties of soil, water, and air. Spectroscopy can be used to identify pollutants in the environment and to monitor the quality of drinking water and industrial wastewater.4. Biomedical ResearchIn biomedical research, spectroscopy is used to study the properties of biological molecules, such as proteins and DNA. Spectroscopy is used to image and diagnose diseases, such as cancer, and to monitor the effectiveness of treatments.ConclusionSpectroscopy and spectral analysis are powerful tools for studying the properties of matter and electromagnetic radiation. There are several types of spectroscopy, each with its own strengths and applications. Spectroscopy and spectral analysis are used in many fields, including chemistry, physics, environmental science, and biomedical research, and have a wide range of applications.。
Bandstructures and Density of States 能带以及态密度
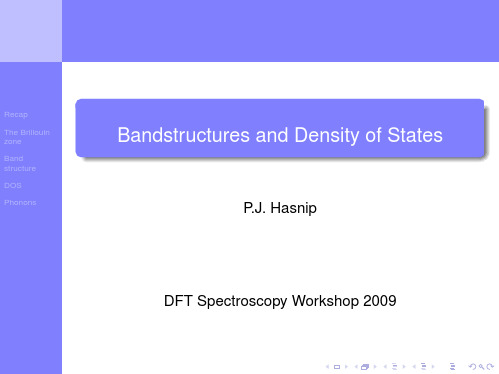
Free Electrons
Recap The Brillouin zone Band structure DOS Phonons
Free Electrons
Recap The Brillouin zone Band structure DOS Phonons
Each state has an energy that changes with k – they form energy bands in reciprocal space. Recall that the energies are periodic in reciprocal-space – there are parabolae centred on each of the reciprocal lattice points.
Free Electrons
Recap The Brillouin zone Band structure DOS Phogs get complicated. In general the reciprocal lattice vectors do not form a simple cubic lattice, and the Brillouin zone can have all kinds of shapes.
First Brillouin Zone (2D)
The region of reciprocal space nearer to the origin than any other allowed wavevector is called the 1st Brillouin zone.
Recap The Brillouin zone Band structure DOS Phonons
spectroscopy 第四讲
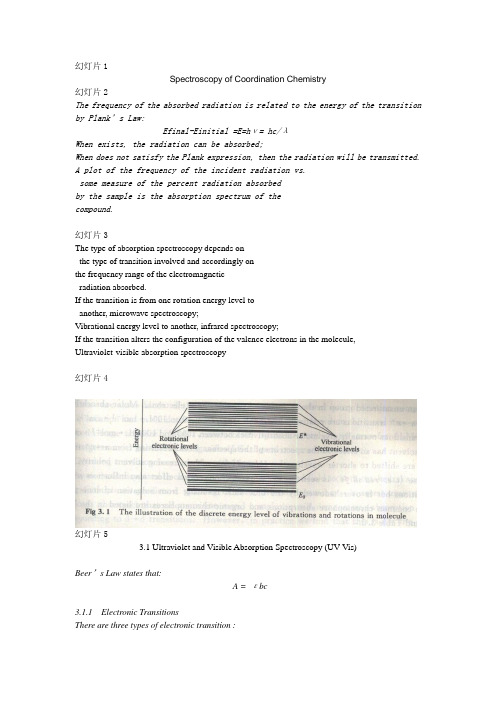
幻灯片1Spectroscopy of Coordination Chemistry幻灯片2The frequency of the absorbed radiation is related to the energy of the transition by Plank’s Law:Efinal-Einitial =E=hν= hc/λWhen exists, the radiation can be absorbed;When does not satisfy the Plank expression, then the radiation will be transmitted.A plot of the frequency of the incident radiation vs.some measure of the percent radiation absorbedby the sample is the absorption spectrum of thecompound.幻灯片3The type of absorption spectroscopy depends onthe type of transition involved and accordingly onthe frequency range of the electromagneticradiation absorbed.If the transition is from one rotation energy level toanother, microwave spectroscopy;Vibrational energy level to another, infrared spectroscopy;If the transition alters the configuration of the valence electrons in the molecule,Ultraviolet-visible absorption spectroscopy幻灯片4幻灯片53.1 Ultraviolet and Visible Absorption Spectroscopy (UV-Vis)Beer’s Law states that:A = εbc3.1.1 Electronic TransitionsThere are three types of electronic transition :1. Transition involving π,σand n electrons;2. charge-transfer electrons3. d and f electrons.幻灯片63.1.2 Absorbing Species Containing π,σand n electronsSince there are superposition of different transition,a continuous absorption band appears.幻灯片7●σσ* Transitions●Transition from a bonding σorbital to the corresponding antibonding orbital (energyis generally large).●CH4 (125 nm) not seen in typical UV-Vis region (200-700 nm).●n σ* Transitions●Saturated compounds containing atoms with lone pairs (non-bonding electrons) arecapable of this type of transitions. In the range of 150-250 nm.●n π* and ππ* Transitions●Most organic compounds have the transitions of n or πelectrons to●the π* excited state and fall in the range of 200-700 nm.●Unsaturated groups providing the πelectrons.●ε= 10 to 100 L·mol-1·cm-1 for n π* transitions.●ε= 1000 to 10000 L·mol-1·cm-1 for ππ* transitions●Solvent effects: blue shift for n π* transitions and red shift for●ππ* transitions with increasing solvent polarity.幻灯片8幻灯片9Charge-Transfer AbsorptionA number of inorganic compounds show charge-transfer absorption.For a complex, if one of its components has electron donating properties and another component can accept electrons.The absorption involves the electron transitions from donor orbital to acceptor orbital. ε> 10000 L·mol-1·cm-1for examples: KMnO4, K2Cr2O7.3.1.3 Electronic Absorption Spectrum of Coordination ComplexThree kinds of electronic transitions: d d transition; MLCT andLMCT(metal-to-ligand charge transfer and ligand-to-metal charge transfer); LC (ligand centered transitions).幻灯片10● d d transitions●According to the selection rules, some transitions are strong (Td●complexes), and others are weak (Oh complexs).●Taking the hydrogen atom as an example:In which the 1s 2p transition is allowed,whereas the 1s 2s transition is “symmetryForbidden”. The reason is that the hydrogen atom possesses a center of inversion.The SALC (Symmetry Adapted Linear Combination)stated that if there is a inversion centre, we require the initial and final states have different parity.Then for a Oh complex, which has an inversion centre, the d d transition is forbidden. Meanwhile, for a Td compound, the d d transition is allowed. Therefore, the d d adsorption intensity of Td complexes is much higher than in Oh complex.We can still observe some d d adsorption in Oh complexes, this is due to the break of Oh symmetry.幻灯片11For example, when a Oh complex vibrate and in some cases the inversion centre does not exist. When this asymmetry is present, a weak absorption is present.This weak relaxation of the Laporte selection rule is known as vibronic(振动) coupling because it arises from the interaction of vibrational modes with the electronic transition modes.This weak absorptions fall in the visible region, which can be used in the explanation of coordination complexes’ colors.(2) MLCT or LMCT transitionThese transitions generally occur in the complexes which involved the metal centered dπground state and ligand π * states and the transitions can be observed in the visible region.For example, for a d6 octahedral metal complex, the molecularorbital diagram is in the following:幻灯片12From the Fig. 3.4, it is clear that the HOMO orbital is predominantlymetal dπorbital based and the LOMO orbital is predominantly ligandπ* orbital based. Normally, π-acceptors ligands will present a low-lying π* orbital and in the same time stabilize the dπorbital centeredon the metal by retro-coordination.Light absorber molecule.幻灯片13The major electronic transition that occur in d6 metal complexeswith unsaturated ligands are ligand based n π*, ππ*, MLCT and LF( ligand field transition) (Fig. 3.5).The intensity of a transition is determined by selection rule.both Laporte and spin rules.(1)Ligand ππ* transition and MLCT are both rules allowed, theεis 103 ~105 L·mol-1·cm-1.(2) LF is spin allowed but Laporte forbidden,εis 102 ~103 L·mol-1·cm-1.幻灯片14The compound [Ru(bpy)3]2+ is a photostable compound(τ=640 nsand emission quantum yield Φ= 0.062). The electronic absorptionspectrum of [Ru(bpy)3]2+ is:幻灯片153.1.4 InstrumentUV-Vis spectrometer:Lamp: a deuterium discharge lamp for UV measurement and a tungsten-halogen lamp for visible and NIR measurements.a normal UV-Vis 190~900 nm. Nitrogen, vacuum detector.幻灯片163.2 Infrared Spectroscopy● 3.2.1 Several types of molecular motion●Translational motion●move through space in some arbitrary direction with a particular velocity.●Rotational motion●rotate about some internal axis.●Vibrational motion●the molecule may vibrate. As shown in Fig. 3.9, a polyatomic molecule has total3N freedom. When abstracting the 3 translational and 3 rotational degrees of freedom, the vibrational freedom is 3N-6.幻灯片17For water molecule, the vibrational freedom is 3*3 –6 = 3.Each of the vibrational motions of a molecule occurs with a certainFrequency, which is characteristic of the molecule and of the particularvibration. The energy involved in particular vibration is characterizedby the amplitude of the vibration, so that the higher the vibrationalEnergy, the larger the amplitude of the motion.Since most vibrational motions in molecules occur at 1014 sec-1. thenLight of wavelength = 3μm will be required to cause transition fromone vibrational energy level to another. This wavelength lies in the so-called infrared region of the spectrum. So, IR spectroscopy deals withvibrational motion of the molecules. Vibrational spectroscopy.幻灯片18● 3.2.2 Application of IR●IR spectrum of organic molecules could be divided into three regions: ●4000 ~ 1300 cm-1 (specific functional groups and bond types);●1300~ 909 cm-1 (the fingerprint region);●909 ~ 605 cm-1( the presence of benzene rings).幻灯片19幻灯片20Here, we consider the IR spectroscopy of inorganic compounds.For example: KNO2, in its lattice, the K+ and NO2- is independently arranged. Therefore, we consider only the NO2- anion (the K+ ionhas no vibrational motion).the nitrite anion has 3 vibrational freedom.One is symmetric stretch at 1335 cm-1,Another is asymmetric stretch at 1250 cm-1,The last one is bending vibration at 830cm-1. and their frequencies are almostSame regardless of the counter ion. So, it can be used for the diagnosis the presence of nitrite ions in a compound.幻灯片21For another salt, NaNO3, it is more complex.For another salt, NaNO3, it is more complex.It should have 3x4-6 = 6 vibrational modes.But its IR spectra exhibit only threeabsorption band centered at 831, 1405 and692 cm-1. it is that the symmetricstretching is not IR active. The reason forthis is that this type of motion gives no rise to the change of thedipole moment of the ion.Among the remaining 5, there are two sets of doubly degeneratevibrations, that is each 2 motions has one band in IR.The IR spectra for some of the more common ions are listed in thefollowing table.幻灯片22幻灯片23The IR absorption bands listed in the above table are for the free ions. When they coordinated with metal ions, the absorption peaks will move. For nitrite anion, it has at least two coordination modes:When coordinated, there willbe an increase of the vibrationalfrequency for the nitrite ionin the order: N-single bondedO (in O-bonded) < NO (in N-bonded) < N-double bond-O(in O-bonded) .幻灯片24In agreement with this, it has been found that in complexes in which NO2- is bonded through oxygen, the two N-O stretching frequencies lie in the ranges 1500~1400 cm-1 for N=O and 1100-1000 cm-1 for N-O.In complexes in which NO2- is bonded through nitrogen, the bands occur at similar frequencies which are intermediate between the range above; namely, 1340~1300 cm-1 and 1430 ~1360 cm-1. Thus it is relatively easy to tell whether a nitrite ion is coordinated through O or N on the basis of IR whether it is coordinated.幻灯片25For nitrate complexes, the nitrate can coordinated with metal atomin the following ways:In the free nitrate ion, thethree oxygen atoms areidentical, but no longeridentical in the coordinatednitrate ion.In all three cases, two of theoxygen atoms are identicaland the third one is unique.we say that the AB3 typeion is to change to an AB2C type species. Then the IR inactive symmetric stretching mode for the free nitrate ion (AB3) type becomes IR active when coordinated to metal atoms(AB2C).幻灯片26Similarly, the doubly degenerated asymmetric stretch in AB3becomes two asymmetric stretch with different energy in AB2C.Free nitrate ion: single band; coordinated nitrate ion: two bands.The more symmetrical a molecules or ion is, the fewer the number of bands that will appearin the IR spectrum.幻灯片27principle of Raman spectroscopyRayleigh散射:弹性碰撞;无能量交换,仅改变方向;Raman散射:非弹性碰撞;方向改变且有能量交换;Rayleigh散射Raman散射E0基态,E1振动激发态;E0 + h0 ,E1 + h0 激发虚态;获得能量后,跃迁到激发虚态.(1928年印度物理学家Raman C V 发现;1960年快速发展)幻灯片28基本原理E0E1 V=1V=0- 激发虚态1. Raman 散射Raman 散射的两种跃迁能量差: E=h(0 - )产生stokes 线;强;基态分子多; E=h(0 + ) 产生反stokes 线;弱; Raman 位移:Raman幻灯片29 2. Raman 位移对不同物质:对同一物质: -转能级的特征物理量;定性与结构分析的依据;Raman 散射的产生:光电场E= E 分子极化率; 幻灯片30E0E1V=1V=0-ANTI-STOKES-RayleighSTOKES诱导偶极矩 = E非极性基团,对称分子;拉曼活性振动—伴随有极化率变化的振动。
第七章星系系统的介绍
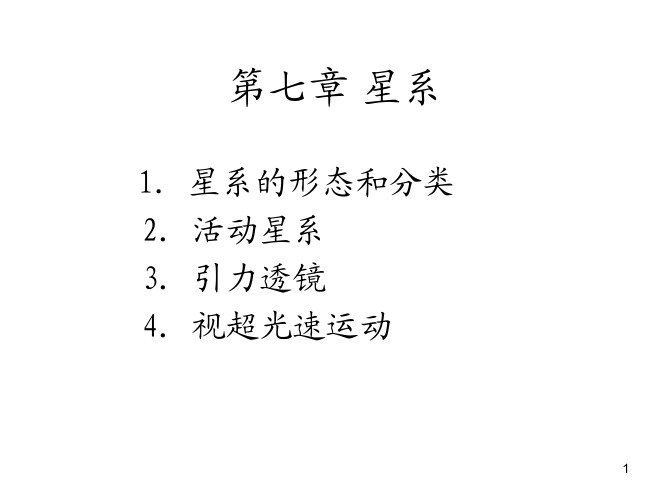
17
棒旋星系 (Barred spiral galaxies)
Type SBa
Type SBb
Type SBc
18
银河系主要成分 (1) 银盘 (disk) (旋臂spiral arm)、 (2) 核球 (bulge) 、 (3) 银晕 (halo) 、(4) 银 冕 (corona)
19
星际物质(星际介质,Interstellar Medium) 星系内分布在恒星与恒星之间(~ 6-10 ly)的物质。 包括星际气体、星际尘埃、宇宙线与星际磁场。 星际物质的质量约为银河系恒星质量的10%。 主要分布在距离银道面约1000 ly的范围内。
33
所有这些激烈的物理过程主要是集中在星系的 核心,或者是由核心引发的。 通常也称这类星系为活动星系核(Active Galactic Nuclei,简称AGN) 只要不是专门讨论活动星系本身的结构,两者 之间不再严格加以区分
34
1918年(美)柯 蒂斯(Curtis)发 现星“云”M87的 光学喷流
28
星系质量测量结果
正常旋涡星系质量~ 109 -1012 M⊙ 椭圆星系质量~ 105 -1013 M⊙ 不规则星系质量~ 106 -1010 M⊙ 星系团质量~ 1013 -1014 M⊙ 星系和星系团的引力质量大约是可见质量的10倍。
29
星系的大小变化很大: 不规则星系,只有银河系的1%-25%; 巨椭圆星系,银河系大小的5倍; 矮椭圆星系,银河系大小的1%。
10
哈勃的裁决
1924年,哈勃 (Edwin Hubble) 分解出 “仙女座大星云” (M31) 中的造父变星, 证实它确实是恒星系统。 由造父变星周光关系哈勃估计M31的距 离285 kpc(实际距离778 kpc) > 最远 的球状星团的距离 (100 kpc) 。
UBVI CCD photometry of two old open clusters NGC 1798 and NGC 2192

a r X i v :a s t r o -p h /9901140v 1 12 J a n 1999Mon.Not.R.Astron.Soc.000,1–8(1998)Printed 1February 2008(MN L A T E X style file v1.4)UBV I CCD photometry of two old open clusters NGC 1798and NGC 2192Hong Soo Park and Myung Gyoon Lee ⋆Department of Astronomy,Seoul National University,Seoul 151-742,KoreaAccepted 1998??.Received 1998??;in original form 1998July ??ABSTRACT We present UBV I CCD photometry of two open clusters NGC 1798and NGC 2192which were little studied before.Color-magnitude diagrams of these clusters show several features typical for old open clusters:a well-defined main-sequence,a red giant clump,and a small number of red giants.The main sequence of NGC 1798shows a distinct gap at V ≈16.2mag.From the surface number density distribution we have measured the size of the clusters,obtaining 8′.3(=10.2pc)for NGC 1798and 7′.3(=7.5pc)for NGC 2192.Then we have determined the reddening,metallicity,and distance of these clusters using the color-color diagrams and color-magnitude diagrams:E (B −V )=0.51±0.04,[Fe/H]=−0.47±0.15dex and (m −M )0=13.1±0.2(d =4.2±0.3kpc)for NGC 1798,and E (B −V )=0.20±0.03,[Fe/H]=−0.31±0.15dex and (m −M )0=12.7±0.2(d =3.5±0.3kpc)for NGC 2192.The ages of these clusters have been estimated using the morphological age indicators and the isochrone fitting with the Padova isochrones:1.4±0.2Gyrs for NGC 1798and 1.1±0.1Gyrs for NGC 2192.The luminosity functions of the main sequence stars in these clusters are found to be similar to other old open clusters.The metallicity and distance of these clusters are consistent with the relation between the metallicity and galactocentric distance of other old open clusters.Key words:Hertzsprung–Russell (HR)diagram –open clusters and associations:general –open clusters and associations:individual:NGC 1798,NGC 2192–stars:luminosity function.1INTRODUCTIONOld open clusters provide us with an important informationfor understanding the early evolution of the Galactic disk.There are about 70known old open clusters with age >1Gyrs (Friel 1995).These clusters are faint in general so thatthere were few studies about these clusters until recently.With the advent of CCD camera in astronomy,the numberof studies on these clusters has been increasing.However,there are still a significant number of old open clusters forwhich basic parameters are not well known.For example,metallicity is not yet known for about 30clusters amongthem.Recently Phelps et al.(1994)and Janes &Phelps (1994)presented an extensive CCD photometric survey of potentialold open clusters,the results of which were used in the studyon the development of the Galactic disk by Janes &Phelps(1994).In the sample of the clusters studied by Phelps et al.⋆corresponding author,E-mail:mglee@astrog.snu.ac.krthere are several clusters for which only the non-calibrated photometry is available.We have chosen two clusters among them,NGC 1798and NGC 2192,to study the characteristics of these clus-ters using UBV I CCD photometry.These clusters are lo-cated in the direction of anti-galactic centre.To date there is published only one photometric study of these clusters,which was given by Phelps et al.(1994)who presented non-calibrated BV CCD photometry of these clusters.From the instrumental color-magnitude diagrams of these clus-ters Phelps et al.estimated the ages of these clusters using the morphological age indicators,obtaining the values of 1.5Gyrs for NGC 1798and 1.1Gyrs for NGC 2192.However,no other properties of these clusters are yet known.In this paper we present a study of NGC 1798and NGC 2192based on UBV I CCD photometry.We have es-timated the basic parameters of these clusters:size,red-dening,metallicity,distance,and age.Also we have derived the luminosity function of the main sequence stars in these clusters.Section 2describes the observations and data re-duction.Sections 3and 4present the analysis for NGC 1798c 1998RAS2Hong Soo Park and Myung Gyoon Leeand NGC2192,respectively.Section5discusses the results. Finally Section6summarizes the primary results.2OBSER V ATIONS AND DATA REDUCTION 2.1ObservationsUBV I CCD images of NGC1798and NGC2192were obtained using the Photometrics512CCD camera at the Sobaeksan Observatory61cm telescope in Korea for several observing runs between1996November and1997October. We have used also BV CCD images of the central region of NGC1798obtained by Chul Hee Kim using the Tek1024 CCD camera at the Vainu Bappu Observatory2.3m tele-scope in India on March4,1998.The observing log is given in Table1.The original CCD images wereflattened after bias sub-traction and several exposures for eachfilter were combined into a single image for further reduction.The sizes of the field in a CCD image are4′.3×4′.3for the PM512CCD im-age,and10′.6×10′.6for the Tek1024CCD image.The gain and readout noise are,respectively,9electrons/ADU and 10.4electrons for the PM512CCD,and9electrons/ADU and10.4electrons for the Tek1024CCD.Figs.1and2illustrate grey scale maps of the V CCD images of NGC1798and NGC2192made by mosaicing the images of the observed regions.It is seen from thesefigures that NGC1798is a relatively rich open cluster,while NGC 2192is a relatively poor open cluster.2.2Data ReductionInstrumental magnitudes of the stars in the CCD images were obtained using the digital stellar photometry reduction program IRAF⋆/DAOPHOT(Stetson1987,Davis1994). The resulting instrumental magnitudes were transformed onto the standard system using the standard stars from Lan-dolt(1992)and the M67stars in Montgomery et al.(1993) observed on the same photometric nights.The transforma-tion equations areV=v+a V(b−v)+k V X+Z V,(B−V)=a BV(b−v)+k BV X+Z BV,(U−B)=a UB(u−b)+k UB X+Z UB,and(V−I)=a V I(v−i)+k V I X+Z V I,where the lower case symbols represent instrumental mag-nitudes derived from the CCD images and the upper case symbols represent the standard system values.X is the air-mass at the midpoint of the observations.The results of the transformation are summarized in Table2.The data ob-tained on non-photometric nights were calibrated using the photometric data for the overlapped region.The total number of the measured stars is1,416for NGC1798and409for NGC2192.Tables3and4list the⋆IRAF is distributed by the National Optical Astronomy Ob-servatories,which are operated by the Association of Universities for Research in Astronomy,Inc.under contract with the National Science Foundation.photometry of the bright stars in the C-regions of NGC1798 and NGC2192,respectively.The X and Y coordinates listed in Table3and4are given in units of CCD pixel(=0′′.50). The X and Y values are increasing toward north and west, respectively.We have divided the entire region of thefields into sev-eral regions,as shown in Figs.1and2,for the analysis of the data.The C-region represents the central region of the cluster,and the F-regions(F,Fb,Fir,and Fi regions)rep-resent the controlfield regions,and the N-region represents the intermediate region between the central region and the field region.The radius of the C-region is300pixel for NGC 1798and NGC2192.The ratio of the areas of the C-region, N-region,Fb-region,and(Fi+Fir)-regions for NGC1798is 1:1.50:1.00:1.07,and the ratio of the areas of the C-region, N-region,and F-region for NGC2192is1:1.26:0.98.3ANALYSIS FOR NGC17983.1The Size of the ClusterWe have investigated the structure of NGC1798using star-counts.The centre of the cluster is estimated to be at the po-sition of(X=710pixel,Y=1110pixel),using the centroid method.Fig.3illustrates the projected surface number den-sity profile derived from counting stars with V<19.5mag in the entire CCDfield.The magnitude cutofffor starcounts was set so that the counts should be free of any photometric incompleteness problem.Fig.3shows that most of the stars in NGC1798are concentrated within the radius of250pixel (=125′′),and that the outskirts of the cluster extend out to about500pixel(=250′′)from the center.The number density changes little with radius beyond500pixel,show-ing that the outer region of the observedfield can be used as a controlfield.Therefore we have estimated the approxi-mate size of NGC1798for which the cluster blends in with thefield to be about500′′in diameter,which corresponds to a linear size of10.2pc for the distance of NGC1798as determined below.3.2Color-Magnitude DiagramsFigs.4and5show the V−(B−V)and V−(V−I)color-magnitude diagrams(CMDs)of the measured stars in the observed regions in NGC1798.Thesefigures show that the C-region consists mostly of the members of NGC1798with some contamination of thefield stars,while the F-regions consist mostly of thefield stars.The N-region is intermediate between the C-region and the F-region.The distinguishable features seen in the color-magnitude diagrams of the C-region are:(a)There is a well-defined main sequence the top of which is located at V≈16 mag;(b)There is seen a distinct gap at V≈16.2mag in the main sequence,which is often seen in other old open clusters (e.g.M67);(c)There is a poorly defined red giant branch and these is seen some excess of stars around(B−V)=1.3 and V=15.6mag on this giant branch,which is remarked by the small box in thefigures.This may be a random excess of stars.However,the positions of the stars in the CMDs are consistent with the positions of known red giant clump in other old open clusters.Therefore most of these stars arec 1998RAS,MNRAS000,1–8UBV I CCD photometry of two old open clusters NGC1798and NGC21923probably red giant clump stars;and(d)There are a small number of stars along the locus of the red giant branch. 3.3Reddening and MetallicityNGC1798is located close to the galactic plane in the anti-galactic centre direction(b=4◦.85and l=160◦.76)so that it is expected that the reddening toward this cluster is significant.We have estimated the reddening for NGC1798 using two methods as follows.First we have used the mean color of the red giant clump.Janes&Phelps(1994)estimated the mean color and magnitude of the red giant clump in old open clusters to be (B−V)RGC=0.87±0.02and M V,RGC=0.59±0.09,when the difference between the red giant clump and the main sequence turn-offof the clusters,δV,is smaller than one. The mean color of the red giant clump in the C-region is estimated to be(B−V)RGC=1.34±0.01((V−I)RGC= 1.47±0.01,and(U−B)RGC=1.62±0.04),and the cor-responding mean magnitude is V RGC=15.57±0.05.δV is estimated to be0.8±0.2,which is the same value derived by Phelps et al.(1994).From these data we have derived a value of the reddening,E(B−V)=0.47±0.02.Secondly we have used the color-color diagram to es-timate the reddening and the metallicity simultaneously. We havefitted the mean colors of the stars in the C-region with the color-color relation used in the Padova isochrones (Bertelli et al.1994).This process requires iteration,because we need to know the age of the cluster as well as the red-dening and metallicity.We have iterated this process until all three parameters are stabilized.Fig.6illustrates the results offitting in the(U−B)−(B−V)color-color diagram.It is shown in thisfigure that the stars in NGC1798are reasonablyfitted by the color-color relation of the isochrones for[Fe/H]=−0.47±0.15 with a reddening value of E(B−V)=0.55±0.05. The error for the metallicity,0.15,was estimated by com-paring isochrones with different metallicities.As a reference the mean locus of the giants for solar abundance given by Schmidt-Kaler(1982)is also plotted in Fig.6.Finally we derive a mean value of the two estimates for the reddening, E(B−V)=0.51±0.04.3.4DistanceWe have estimated the distance to NGC1798using two methods as follows.First we have used the mean magnitude of the red giant clump.We have derived a value of the ap-parent distance modulus(m−M)V=14.98±0.10from the values for the mean magnitudes of the red giant clump stars described above.Secondly we have used the the zero-age main sequence (ZAMS)fitting,following the method described in Vanden-Berg&Poll(1989).VandenBerg&Poll(1989)presented the semi-empirical ZAMS as a function of the metallicity[Fe/H] and the helium abundance Y:V=M V(B−V)+δM V(Y)+δM V([Fe/H])whereδM V(Y)=2.6(Y−0.27)andδM V([Fe/H])=−[Fe/H](1.444+0.362[Fe/H]).Before the ZAMSfitting,we subtracted statistically the contribution due to thefield stars in the CMDs of the C-region using the CMDs of the Fb-region for BV photometry and the CMDs of the Fi+Fir region for V I photometry. The size of the bin used for the subtraction is∆V=0.25 and∆(B−V)=0.1.The resulting CMDs are displayed in Fig.7.We used the metallicity of[Fe/H]=–0.47as derived above and adopted Y=0.28which is the mean value for old open clusters(Gratton1982).Using this method we have obtained a value of the apparent distance modulus(m−M)V=14.5±0.2.Finally we calculate a mean value of the two estimates,(m−M)V=14.7±0.2.Adopting the extinction law of A V=3.2E(B−V),we derive a value of the intrinsic distance modulus(m−M)0=13.1±0.2.This corresponds to a distance of d=4.2±0.3kpc.3.5AgeWe have estimated the age of NGC1798using two methods as follows.First we have used the morphological age index (MAI)as described in Phelps et al.(1994).Phelps et al. (1994)and Janes&Phelps(1994)presented the MAI–δV relation,MAI[Gyrs]=0.73×10(0.256δV+0.0662δV2).From the value ofδV derived above,0.8±0.2mag,we obtain a value for the age,MAI=1.3±0.2Gyrs.Secondly we have estimated the age of the cluster us-ing the theoretical isochrones given by the Padova group (Bertelli et al.1994).Fitting the isochrones for[Fe/H]=–0.47to the CMDs of NGC1798,as shown in Fig.8,we estimate the age to be1.4±0.2Gyrs.Both results agree very well.3.6Luminosity FunctionWe have derived the V luminosity functions of the main sequence stars in NGC1798,which are displayed in Fig.9.The Fb-region was used for subtraction of thefield star contribution from the C-region and the magnitude bin size used is0.5mag.This controlfield may not be far enough from the cluster to derive thefield star contribution.If so,we might have oversubtracted thefield contribution,obtaining flatter luminosity functions than true luminosity functions. However,the fraction of the cluster members in thisfield must be,if any,very low,because the surface number density of this region is almost constant with the radius as shown in Fig.3.The luminosity function of the C-region in Fig. 9(a)increases rapidly up to V≈16.5mag,and stays almost flat for V>16.5mag.The luminosity functions of the N-region and the(R+Fir)-region are steeper than that of the C-region.A remarkable drop is seen at V=16.2mag(M V= 1.5mag)in the luminosity function of the C-region based on smaller bin size of0.2mag in Fig.9(b).This corresponds to the main sequence gap described above.4ANALYSIS FOR NGC21924.1The Size of the ClusterWe have investigated the structure of NGC2192using star-counts.We could not use the centroid method to estimatec 1998RAS,MNRAS000,1–84Hong Soo Park and Myung Gyoon Leethe centre of this cluster,because this cluster is too sparse. So we have used eye-estimate to determine the centre of the cluster to be at the position of(X=465pixel,Y=930 pixel).Fig.10illustrates the projected surface number den-sity profile derived from counting stars with V<18mag in the entire CCDfield.The magnitude cutofffor starcounts was set so that the counts should be free of any photomet-ric incompleteness problem.Fig.10shows that most of the stars in NGC2192are concentrated within the radius of200 pixel(=100′′),and that the outskirts of the cluster extend out to about440pixel(=220′′)from the centre.Therefore the approximate size of NGC2192is estimated to be about 440′′in diameter,which corresponds to a linear size of7.5pc for the distance of NGC2192as determined below.4.2Color-Magnitude DiagramsFigs.11and12show the V−(B−V)and V−(V−I)color-magnitude diagrams of the measured stars in the observed regions in NGC2192.The distinguishable features seen in the color-magnitude diagrams of the C-region are:(a)There is a well-defined main sequence the top of which is located at V≈14mag;(b)There are a group of red giant clump stars at(B−V)=1.1and V=14.2mag,which are remarked by the small box in thefigures;and(c)There are a small number of stars along the locus of the red giant branch. 4.3Reddening and MetallicityNGC2192is located11degrees above the galactic plane in the anti-galactic centre direction(b=10◦.64and l= 173◦.41)but higher than NGC1798so that it is expected that the reddening toward this cluster is significant but smaller than that of NGC1798.We have estimated the red-dening for NGC2192using two methods as applied for NGC 1798.First we have used the mean color of the red giant clump.The mean color of the red giant clump in the C-region is estimated to be(B−V)RGC=1.08±0.01((V−I)RGC=1.07±0.01,and(U−B)RGC=0.61±0.02),and the corresponding mean magnitude is V RGC=14.20±0.05.δV is estimated to be0.6±0.2,which is similar to the value derived by Phelps et al.(1994).From these data we have derived a value of the reddening,E(B−V)=0.19±0.03.Secondly we have used the color-color diagram to es-timate the reddening and the metallicity simultaneously. We havefitted the mean colors of the stars in the C-region with the color-color relation used in the Padova isochrones (Bertelli et al.1994).Fig.13illustrates the results offit-ting in the(U−B)−(B−V)color-color diagram.It is shown in thisfigure that the stars in NGC2192are rea-sonablyfitted by the color-color relation of the isochrones for[Fe/H]=−0.31±0.15dex with a reddening value of E(B−V)=0.21±0.01.The error for the metallicity,0.15, was estimated by comparing isochrones with different metal-licities.As a reference the mean locus of the giant for solar abundance given by Schmidt-Kaler is also plotted in Fig.13. Finally we derive a mean value of the two estimates for the reddening,E(B−V)=0.20±0.03.4.4DistanceWe have estimated the distance to NGC2192using two methods as for NGC1798.First we have used the mean magnitude of the red giant clump.We have derived a value of the apparent distance modulus(m−M)V=13.61±0.10 from the values for the mean magnitudes of the red giant clump stars described previously.Secondly we have used the ZAMSfitting.Before the ZAMSfitting,we subtracted statistically the contribution due to thefield stars in the CMDs of the C-region using the CMDs of the F-region.The size of the bin used for the subtraction is∆V=0.25and∆(B−V)=0.1.The resulting CMDs are displayed in Fig.14.We used the metallicity of[Fe/H]=–0.31as derived before and adopted Y=ing this method we have obtaineda value of the apparent distance modulus(m−M)V=13.1±0.2.Finally we calculate a mean value of the two estimates,(m−M)V=13.3±0.2.Adopting the extinction law of A V=3.2E(B−V),we derive a value of the intrinsic distance modulus(m−M)0=12.7±0.2.This corresponds to a distance of d=3.5±0.3kpc.4.5AgeWe have estimated the age of NGC2192using two methods as follows.First we have used the morphological age index. From the value ofδV derived above,0.6±0.2mag,we obtain a value for the age,MAI=1.1±0.2Gyrs.Secondly we have estimated the age of the cluster using the theoretical isochrones given by the Padova group(Bertelli et al.1994). Fitting the isochrones for[Fe/H]=–0.31to the CMDs of NGC2192,as shown in Fig.15,we estimate the age to be 1.1±0.1Gyrs.Both results agree very well.4.6Luminosity FunctionWe have derived the V luminosity functions of the main sequence stars in NGC2192,which are displayed in Fig.16.The F-region was used for subtraction of thefield star contribution from the C-region.The luminosity function of the C-region in Fig.16(a)increases rapidly up to V≈14 mag,and stays almostflat for V>15mag.The luminosity function of the N-region is steeper than that of the C-region. Fig.16(b)displays a comparison of the luminosity functions of NGC1798,NGC2192,and NGC7789which is another old open cluster of similar age(Roger et al.1994).Fig.16(b) shows that the luminosity functions of these clusters are similar in that they are almostflat in the faint part.The flattening of the faint part of the luminosity functions of old open clusters has been known since long,and is believed to be due to evaporation of low mass stars(Friel1995).5DISCUSSIONWe have determined the metallicity and distance of NGC 1798and NGC2192in this study.We compare them with those of other old open clusters here.Fig.17illustrates the radial metallicity gradient of the old open clusters com-piled by Friel(1995)and supplemented by the data in Wee&Lee(1996)and Lee(1997).Fig.17shows that thec 1998RAS,MNRAS000,1–8UBV I CCD photometry of two old open clusters NGC1798and NGC21925 mean metallicity decreases as the galactocentric distance in-creases.The positions of NGC1798and NGC2192we haveobtained in this study are consistent with the mean trendof the other old open clusters.The slope we have deter-mined for the entire sample including these two clusters is∆[Fe/H]/R GC=−0.086±0.011dex/kpc,very similar tothat given in Friel(1995),∆[Fe/H]/R GC=−0.091±0.014dex/kpc.There are only four old open clusters located beyondR GC=13kpc in Fig.17.These four clusters follow the meantrend of decreasing outward.However,the number of theclusters is not large enough to decide whether the metallictykeeps decreasing outward or it stops decreasing somewherebeyond R GC=13kpc and stays constant.Further studiesof more old open clusters beyond R GC=13kpc are neededto investigate this point.6SUMMARY AND CONCLUSIONSWe have presented UBV I photometry of old open clustersNGC1798and NGC2192.From the photometric data wehave determined the size,reddening,metallicity,distance,and age of these clusters.The luminosity functions of themain sequence stars in these clusters are similar to those ofthe other old open clusters.The basic properties of theseclusters we have determined in this study are summarizedin Table5.ACKNOWLEDGMENTSProf.Chul Hee Kim is thanked for providing the BV CCDimages of NGC1798.This research is supported in part bythe Korea Science and Engineering Foundation Grant No.95-0702-01-01-3.REFERENCESBertelli,G.,Bressan,A.,Chiosi,C.,Fagotto,F.,&Nasi,E.1994,A&AS,106,275Davis,L.E.,1994,A Reference Guide to the IRAF/DAOPHOTPackageFriel,E.D.1995,ARA&A,33,381Gratton,R.G.,1982,ApJ,257,640Janes,K.,&Phelps,R.L.1994,AJ,108,1773Landolt,A.U.,1992,AJ,104,340Lee,M.G.,1997,113,729Montgomery,K.A.,Marschall,L.A.,&Janes,K.A.1993,AJ,106,181Phelps,R.L.,Janes,K.,&Montgomery,K.A.,1994,AJ,107,1079Roger,C.M.,Paez,E.,Castellani,V.,&Staniero,O.1994,A&A,290,62Schmidt-Kaler,T.1982,in Landolt-Bornstein VI,2b(Berlin:Springer)Stetson,P.B.,1987,PASP,99,191VandenBerg,D.A.,&Poll,H.E.,1989,AJ,98,1451Wee,S.O.,&Lee,M.G.,1996,Jour.Korean Astro.Soc.,29,181This paper has been produced using the Royal AstronomicalSociety/Blackwell Science L A T E X stylefile.c 1998RAS,MNRAS000,1–86Hong Soo Park and Myung Gyoon LeeTable2.Transformation coefficients for the standard stars.Date Color a k Z rms n(stars) 96.11.11V0.003–0.101–6.0400.00919(B−V) 1.090–0.155–0.4670.01317(U−B) 1.008–0.154–1.7110.03416 97.02.11V0.028–0.198–6.0500.00934(B−V) 1.150–0.118–0.5930.01035(U−B) 1.079–0.324–1.5750.03226(V−I)0.983–0.1300.3020.00829 97.02.13V–0.007–0.176–6.0050.01034(B−V) 1.160–0.133–0.5880.01334(U−B) 1.079–0.349–1.5260.02320(V−I)0.986–0.0970.2710.01532 97.03.17V-0.019–0.225–6.0680.01548(B−V) 1.221–0.093–0.7810.01844(U−B) 1.008–0.309–1.4580.02735(V−I)0.956–0.1570.3140.02052Table 3.UBV I photometry of the bright stars in the C-regionof NGC1798.ID X[px]Y[px]V(B−V)(U−B)(V−I) 1723.21110.815.8600.7790.3240.9143680.51092.015.380 1.3250.627 1.5254746.01160.315.9050.7410.2200.9545741.81165.514.380 1.4830.880 1.6336684.61030.115.8140.8640.342 1.0427745.31073.315.3760.9200.237 1.0849707.21038.814.777 1.4860.723 1.653 11549.41153.715.305 1.5290.915 1.666 12547.91162.714.846 1.6270.947 1.764 13602.31068.015.325 1.5240.847 1.660 14598.11080.815.776 1.3340.575 1.490 15525.81116.815.510 1.0020.346 1.169 18833.91144.214.976 1.4940.866 1.578 21981.91125.114.711 1.510 1.031 1.440 26835.71098.015.706 1.3180.468 1.407 27656.51022.115.653 1.4160.180 1.571 28659.8955.915.216 1.2160.384 1.385 29656.4976.813.6670.6610.3100.777 30674.2822.512.0330.4450.0500.532 33668.7966.315.687 1.3130.641 1.446 34764.3879.915.460 1.3370.508 1.408 38749.01008.115.359 1.3220.744 1.426 39774.4965.915.545 1.3040.590 1.402 43571.8930.612.875 1.970 1.264 2.252 45512.8908.715.810 1.3480.474 1.485 46642.1949.515.622 1.3290.590 1.477 48732.61211.315.821 1.3530.643 1.492 50678.81210.515.297 1.3680.770 1.501 51742.11244.414.7990.747-0.046 1.216 52673.11250.215.180 1.3060.780 1.457 56728.21183.415.665 1.4050.803 1.545 57614.91229.413.085 2.1770.635 3.581 62563.01338.415.9090.7060.6380.778 65913.91203.013.686 1.2490.851 1.354 67792.71314.215.6010.9750.470 1.275 68932.61268.414.4660.7170.1970.763 70801.11338.715.561 1.3270.785 1.442 72827.61315.513.5260.9670.521 1.161 78444.01094.814.605 1.5790.825 1.723 82684.3822.615.8280.9510.128 1.189 97598.51330.315.580 1.3360.710 1.483Table4.UBV I photometry of the bright stars in the C-region of NGC2192.ID X[px]Y[px]V(B−V)(U−B)(V−I) 4502.4879.614.9830.4320.1140.424 5537.5891.712.9690.9240.4750.930 7535.1931.315.1700.598-0.2010.483 9434.8947.613.6760.570-0.0560.648 10562.3991.914.037 1.0960.612 1.043 11412.9884.814.165 1.0780.608 1.072 12598.4778.715.1840.464-0.0300.477 13595.9930.014.4800.5020.1720.549 14625.8971.814.7430.7200.0890.741 16614.3684.214.3970.7660.1750.758 17463.3689.514.7660.4660.0120.480 18553.3710.715.4920.447-0.1350.428 19591.7723.814.4660.5450.0930.566 21623.0867.712.634 1.1020.713 1.108 22648.0929.015.3380.3940.1150.399 26461.1711.314.162 1.0860.670 1.091 29583.9802.315.0940.600-0.0160.644 32720.4889.515.3260.3700.0710.410 37395.9778.315.2640.3960.0310.379 38405.5778.514.8900.4810.1010.409 40258.21042.915.4310.4240.1200.484 42376.6883.915.1870.4550.1360.470 44180.1908.714.3200.2870.1120.295 47367.1938.314.8970.4030.2520.459 51183.3998.714.202 1.1140.552 1.108 55475.81052.514.6370.3880.1670.465 56389.11055.113.6060.6520.2560.777 58481.41074.114.3470.4500.1150.520 59514.91085.115.0970.4290.1370.517 60478.51089.614.2360.5280.1590.582 61498.51127.914.6090.5090.1350.578 63278.71154.414.372 1.0700.599 1.053 64527.41158.715.4180.4460.2450.510 65343.41167.814.266 1.0120.618 1.035 66362.21169.314.2660.8790.4690.879 74346.91039.814.5810.4520.1240.510 77688.91124.215.4200.3900.2900.400 83678.91079.715.4950.4640.1070.504 84624.21042.813.5010.3850.2170.389 88579.61028.914.8250.4560.1620.530 Table5.Basic properties of NGC1798and NGC2192.Parameter NGC1798NGC2192RA(2000)5h11m40s6h15m11sDEC(2000)47◦40′37′′39◦51′1′′l160◦.76173◦.41b4◦.8510◦.64Age1.4±0.2Gyrs1.1±0.1GyrsE(B−V)0.51±0.040.20±0.04[Fe/H]−0.47±0.15dex−0.31±0.15dex(m−M)013.1±0.212.7±0.2distance4.2±0.3kpc3.5±0.3kpcR GC12.5kpc11.9kpcz355pc646pcdiameter10.2pc(8′.3)7.5pc(7′.3)c 1998RAS,MNRAS000,1–8UBV I CCD photometry of two old open clusters NGC1798and NGC21927 Table1.Observing log for NGC1798and NGC2192.Date Target Filter Seeing Telescope Condition96.11.11NCC1798UBV2′′.3SAO a-61cm Photometric97.01.12NCC1798UBV I2′′.2SAO-61cm Non-photometric97.02.12NCC1798,NGC2192UBV I3′′.3SAO-61cm Photometric97.02.13NCC1798,NGC2192UBV I2′′.5SAO-61cm Non-photometric97.03.17NCC2192UBV I2′′.2SAO-61cm Photometric97.10.21NCC1798BV I2′′.3SAO-61cm Non-photometric97.10.24NCC2192BV I2′′.7SAO-61cm Non-photometric97.03.04NCC1798BV2′′.7VBO b-2.3m Non-photometrica Sobaeksan Astronomical Observatoryb Vainu Bappu Observatoryc 1998RAS,MNRAS000,1–8。
拉曼光谱raman Spectroscopy

Histadine300028002600
2400
2200
Wavenumber
2000
Series2 1800
1600
1400
1200
1
101
201
301
401
501
601
701
801
901
1001
1101
1201
1301
1401
1501
1601
1701
1000 1801
Wavelength
Citations And References
Sir Chandrashekhara Venkata Raman
November 7, 1888November 21, 1970 Won the Noble Prize in 1930 for Physics Discovered the "Raman Effect" Besides Discovering the Raman Effect, He studied extensively in X-Ray Diffractions, Acoustics, Optics, Dielectrics, Ultrasonics, Photo electricity, and colloidal particles.
Raman Spectroscopy
Anatasia Gangadin Dr. Ronald Birke City College of New York
What is Spectroscopy?
The study of how 'species' (i.e., atoms, molecules, solutions) react to light. Some studies depend on how much light an atom absorbs. The electromagnetic radiation absorbed, emitted or scattered by the molecule is analyzed. Typically, a beam of radiation from a source such as a laser is passed through a sample, and the radiation exiting the sample is measured. Some, like Raman, depend on a molecule's vibrations in reaction to the light.
核磁共振基本原理与实验操作指导说明书

Chapter 1: NMR Coupling ConstantsNMR can be used for more than simply comparing a product to a literature spectrum. There is a great deal of information that can be learned from analysis of the coupling constants for a compound.1.1Coupling Constants and the Karplus EquationWhen two protons couple to each other, they cause splitting of each other’s peaks. The spacing between the peaks is the same for both protons, and is referred to as the coupling constant or J constant. This number is always given in hertz (Hz), and is determined by the following formula:J Hz = ∆ ppm x instrument frequency∆ ppm is the difference in ppm of two peaks for a given proton. The instrument frequency is determined by the strength of the magnet, and will always be 300 MHz for all spectra collected on the organic teaching lab NMR.Figure 1-1 below shows the simulated NMR spectrum of 1,1-dichloroethane, collected in a 30 MHz instrument. This compound has coupling between A (the quartet at 6 ppm) and B (the doublet at 2 ppm).Figure 1-1: The NMR spectrum of 1,1-dichloroethane, collected in a 30 MHz instrument. For both A and B protons, the peaks are spaced by 0.2 ppm, equal to 6 Hz in this instrument.For both A and B, the distance between the peaks is equal. In this example, the spacing between the peaks is 0.2 ppm (for example, the peaks for A are at 6.2, 6.0, 5.8, and 5.6 ppm). This is equal to a J constant of (0.2 ppm • 30 MHz) = 6 Hz. Since the shifts are given in ppm or parts per million, you should divide by 106. But since the frequency is in megahertz instead of hertz, you should multiply by 106. These two factors cancel each other out, making calculations nice and simple.Figure 1-2 below shows the NMR spectrum of the same compound, but this time collected in a 60 MHz instrument.Chapter 1: NMR Coupling ConstantsFigure 1-2: The NMR spectrum of 1,1-dichloroethane, collected in a 60 MHz instrument. For both A and B protons, the peaks are spaced by 0.1 ppm, equal to 6 Hz in this instrument.This time, the peak spacing is 0.1 ppm. This is equal to a J constant of (0.1 ppm • 60 MHz) = 6 Hz, the same as before. This shows that the J constant for any two particular protons will be the same value in hertz, no matter which instrument is used to measure it.The coupling constant provides valuable information about the structure of a compound. Some typical coupling constants are shown here.Figure 1-3: The coupling constants for some typical pairs of protons.In molecules where the rotation of bonds is constrained (for instance, in double bonds or rings), the coupling constant can provide information about stereochemistry. The Karplus equation describes how the coupling constant between two protons is affected by the dihedral angle between them. The equation follows the general format of J = A + B (cos θ) + C (cos 2θ), with the exact values of A, B and C dependent on several different factors. In general, though, a plot of this equation has the shape shown in Figure 1-4. Coupling constants will usually, but not always, fall into the shaded band on this graph.Figure 1-4: The plot of dihedral angle vs. coupling constant described by the Karplus equation.Chapter 1: NMR Coupling ConstantsThe highest coupling constants will occur between protons that have a dihedral angle of either 0° or 180°, and the lowest coupling constants will occur at 90°. This is due to orbital overlap – when the orbitals are at 90°, there is very little overlap between them, so the hydrogens cannot affect each other’s spins very much (Figure 1-5).Figure 1-5: The best orbital overlap occurs at 180° or 0°, which is why the coupling constant is higher for those angles.1.2 Calculating Coupling Constants in MestreNovaTo calculate coupling constants in MestreNova, there are several options. The easiest one is to use the Multiplet Analysis tool. To do this, go to Analysis → Multiplet Analysis → Manual (or just hit the “J” key). Drag a box around each group of equivalent protons. A purple version of the integral bar will appear below each one, along with a purple box above each one describing its splitting pattern and location in ppm. As with normal integrals, you can right-click the integral bar, select “Edit Multiplet”, and set these integrals to whatever makes sense for that particular structure. For example, in Figure 1-6, each peak is from a single proton so each integral should be about 1.00.Figure 1-6: An example NMR spectrum with multiplet analysis.HH H H HHChapter 1: NMR Coupling ConstantsOnce all peaks are labeled, you can go to Analysis → Multiplet Analysis → Report Multiplets. A text box should appear containing information about the peaks in a highly compressed format. You can then copy and paste this text into your lab report as needed. The spectrum shown above has the following multiplets listed:1H NMR (300 MHz, Chloroform-d) δ 5.14 (d, J = 11.7 Hz, 1H), 4.98 (d, J = 11.7 Hz, 1H), 4.75 (d, J = 3.2 Hz, 1H), 3.37 (d, J = 8.5 Hz, 1H), 3.30 (dd, J = 8.5, 3.3 Hz, 1H).The first set of parentheses indicates that the sample was dissolved in Chloroform-d and placed in a 300 MHz instrument. After that, there is a list of numbers. Each number or range indicates the chemical shift of each of the peaks in the spectrum, in order of descending chemical shift. Each number also has a set of parentheses after it, giving information about that peak. These parentheses contain: • A letter or letters to indicate the splitting of a peak (s=singlet, d=doublet, t=triplet, q=quartet); it is also possible to see things like dd for a doublet of doublets or b for broad. If MestreNova can’t identify a uniform splitting pattern, it will name it a multiplet (m).•The coupling constants or J-values for that peak – for example, the peak at 3.30 ppm has J-values of 8.5 and 3.3 ppm.•The integral of the peak, rounded to the nearest whole number of H.Using this information, you can determine which peaks in Figure 1-6 are coupling to each other based on which ones have matching J-values.•Peaks A and B in Figure 1-6 both have J-values of 11.7 Hz, so these two protons are coupling to each other.•Peaks C and E both have J-values of 3.2 or 3.3 Hz (similar enough, within a margin of error), so these two protons are coupling to each other.•Peaks D and E both have J-values of 8.5 Hz, so these two protons are coupling to each other. If the multiplet analysis tool is failing to determine J-values for any reason, you can always calculate them manually. To do this, you will need to get more precise values for your peak locations. Right-click anywhere in the empty space of the spectrum and select Properties, then go to Peaks and increase the decimals to 4 (Figure 1-7).Chapter 1: NMR Coupling ConstantsFigure 1-7: Changing the decimals on peak labeling.Now if you do peak-picking to label the locations of the peaks, you should see them to 4 decimal places. This will allow you to plus these into the equation to find the J-values manually. For example, in Figure 1-8, the peaks around 4.7 ppm have a J-value of (4.7550 ppm – 4.7442 ppm) • 300 MHz = 3.24 Hz. Note that this in in agreement with MestreNova’s determination of 3.2 ppm for this J-value in Figure1-6.Figure 1-8: Peaks labeled with enough precision to allow you to calculate J-values manually.Chapter 1: NMR Coupling Constants1.3 Topicity and Second-Order CouplingDuring the NMR tutorial, you learned about the concept of chemical equivalence: protons in identical chemical environments have identical chemical shifts. However, just because two protons have the same connectivity to the molecule does not mean they are chemically equivalent. This is related to the concept of topicity : the stereochemical relationship between different groups in a molecule. To find the topicity relationship of two groups to each other, you should try replacing first one group, then the other group with a placeholder atom (in the examples in Figure 1-9, a dark circle is used as the placeholder). If the two molecules produced are identical, then the groups are homotopic; if the molecules are enantiomers, then the groups are enantiotopic; and if the molecules are diastereomers, then the groups are diastereotopic. Groups that are diastereotopic are chemically inequivalent, so they will have a different chemical shift from each other in NMR, and will show coupling as if they were neighboring protons instead of on the same carbon atom.Figure 1-9: Some examples of homotopic, enantiotopic, and diastereotopic groups.If two signals are coupled to each other and have very similar (but not identical) chemical shifts, another effect will appear: second-order coupling. This means that the peaks appear to “lean” toward each other – the peaks on the outside of the coupled pair are shorter, and the peaks on the inside are taller. (Figure 1-10).Figure 1-10: As the chemical shifts of H a and H b become more and more similar, the coupling between them becomes more second-order and the peaks lean more.Chapter 1: NMR Coupling Constants This is very common for two diastereotopic protons on the same carbon atom, but it appears in other situations where two protons are almost chemically identical as well. In Figure 1-8, note the two doublets at 4.98 and 5.14 ppm. These happen to be diastereotopic protons – they are attached to the same carbon, but are chemically equivalent.Looking for pairs of leaning peaks is useful, because it allows you to identify which protons are coupled to each other in a complicated spectrum. In Figure 1-11, there are two different pairs of leaning peaks: two 1H peaks with a J = 9 Hz, and two 2H peaks with J = 15 Hz. Recognizing this makes it possible to pick apart the different components of the peaks towards the left of the spectrum: these are two overlapping doublets, not a quartet.Figure 1-11: An NMR spectrum with two different pairs of leaning peaks.The multiplet tool in MestreNova might not work immediately for analyzing overlapping multiplets like this. Instead, you should follow the instructions at /resolving-overlapped-multiplets/ to deal with them.。
219316026_牛蒡中性多糖结构分析与抗炎活性评价
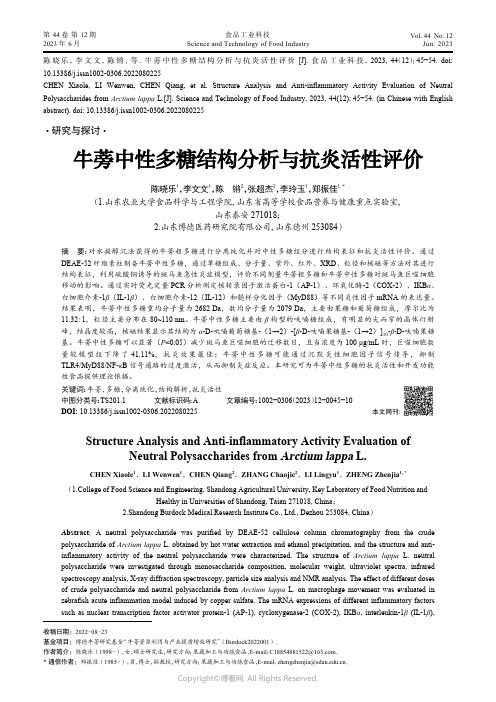
陈晓乐,李文文,陈锵,等. 牛蒡中性多糖结构分析与抗炎活性评价[J]. 食品工业科技,2023,44(12):45−54. doi:10.13386/j.issn1002-0306.2022080225CHEN Xiaole, LI Wenwen, CHEN Qiang, et al. Structure Analysis and Anti-inflammatory Activity Evaluation of Neutral Polysaccharides from Arctium lappa L.[J]. Science and Technology of Food Industry, 2023, 44(12): 45−54. (in Chinese with English abstract). doi: 10.13386/j.issn1002-0306.2022080225· 研究与探讨 ·牛蒡中性多糖结构分析与抗炎活性评价陈晓乐1,李文文1,陈 锵2,张超杰2,李玲玉1,郑振佳1,*(1.山东农业大学食品科学与工程学院,山东省高等学校食品营养与健康重点实验室,山东泰安 271018;2.山东博德医药研究院有限公司,山东德州 253084)摘 要:对水提醇沉法获得的牛蒡粗多糖进行分离纯化并对中性多糖组分进行结构表征和抗炎活性评价。
通过DEAE-52纤维素柱制备牛蒡中性多糖,通过单糖组成、分子量、紫外、红外、XRD 、粒径和核磁等方法对其进行结构表征,利用硫酸铜诱导的斑马鱼急性炎症模型,评价不同剂量牛蒡粗多糖和牛蒡中性多糖对斑马鱼巨噬细胞移动的影响。
通过实时荧光定量PCR 分析测定核转录因子激活蛋白-1(AP-1)、环氧化酶-2(COX-2)、IKB α、白细胞介素-1β(IL-1β)、白细胞介素-12(IL-12)和髓样分化因子(MyD88)等不同炎性因子mRNA 的表达量。
Keck spectroscopy of globular clusters in the spiral galaxy NGC 2683
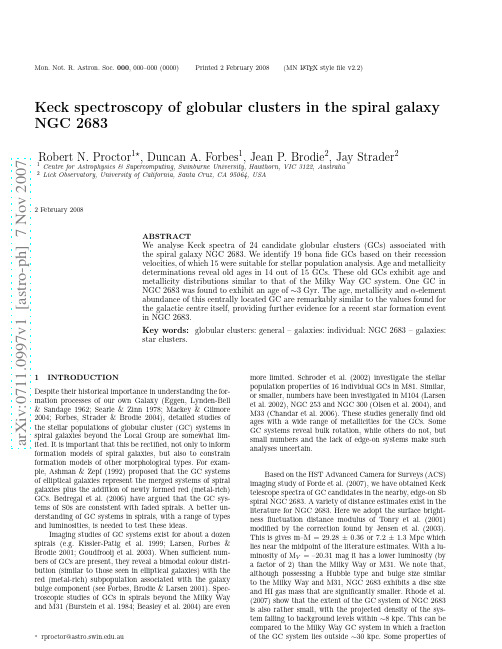
a r X i v :0711.0997v 1 [a s t r o -p h ] 7 N o v 2007Mon.Not.R.Astron.Soc.000,000–000(0000)Printed 2February 2008(MN L A T E X style file v2.2)Keck spectroscopy of globular clusters in the spiral galaxyNGC 2683Robert N.Proctor 1⋆,Duncan A.Forbes 1,Jean P.Brodie 2,Jay Strader 21Centre for Astrophysics &Supercomputing,Swinburne University,Hawthorn,VIC 3122,Australia 2Lick Observatory,University of California,Santa Cruz,CA 95064,USA2February 2008ABSTRACTWe analyse Keck spectra of 24candidate globular clusters (GCs)associated with the spiral galaxy NGC 2683.We identify 19bona fide GCs based on their recession velocities,of which 15were suitable for stellar population analysis.Age and metallicity determinations reveal old ages in 14out of 15GCs.These old GCs exhibit age and metallicity distributions similar to that of the Milky Way GC system.One GC in NGC 2683was found to exhibit an age of ∼3Gyr.The age,metallicity and α-element abundance of this centrally located GC are remarkably similar to the values found for the galactic centre itself,providing further evidence for a recent star formation event in NGC 2683.Key words:globular clusters:general –galaxies:individual:NGC 2683–galaxies:star clusters.1INTRODUCTIONDespite their historical importance in understanding the for-mation processes of our own Galaxy (Eggen,Lynden-Bell &Sandage 1962;Searle &Zinn 1978;Mackey &Gilmore 2004;Forbes,Strader &Brodie 2004),detailed studies of the stellar populations of globular cluster (GC)systems in spiral galaxies beyond the Local Group are somewhat lim-ited.It is important that this be rectified,not only to inform formation models of spiral galaxies,but also to constrain formation models of other morphological types.For exam-ple,Ashman &Zepf (1992)proposed that the GC systems of elliptical galaxies represent the merged systems of spiral galaxies plus the addition of newly formed red (metal-rich)GCs.Bedregal et al.(2006)have argued that the GC sys-tems of S0s are consistent with faded spirals.A better un-derstanding of GC systems in spirals,with a range of types and luminosities,is needed to test these ideas.Imaging studies of GC systems exist for about a dozen spirals (e.g.Kissler-Patig et al.1999;Larsen,Forbes &Brodie 2001;Goudfrooij et al.2003).When sufficient num-bers of GCs are present,they reveal a bimodal colour distri-bution (similar to those seen in elliptical galaxies)with the red (metal-rich)subpopulation associated with the galaxy bulge component (see Forbes,Brodie &Larsen 2001).Spec-troscopic studies of GCs in spirals beyond the Milky Way and M31(Burstein et al.1984;Beasley et al.2004)are even⋆rproctor@.aumore limited.Schroder et al.(2002)investigate the stellar population properties of 16individual GCs in M81.Similar,or smaller,numbers have been investigated in M104(Larsen et al.2002),NGC 253and NGC 300(Olsen et al.2004),and M33(Chandar et al.2006).These studies generally find old ages with a wide range of metallicities for the GCs.Some GC systems reveal bulk rotation,while others do not,but small numbers and the lack of edge-on systems make such analyses uncertain.Based on the HST Advanced Camera for Surveys (ACS)imaging study of Forde et al.(2007),we have obtained Keck telescope spectra of GC candidates in the nearby,edge-on Sb spiral NGC 2683.A variety of distance estimates exist in the literature for NGC 2683.Here we adopt the surface bright-ness fluctuation distance modulus of Tonry et al.(2001)modified by the correction found by Jensen et al.(2003).This is gives m–M =29.28±0.36or 7.2±1.3Mpc which lies near the midpoint of the literature estimates.With a lu-minosity of M V =–20.31mag it has a lower luminosity (by a factor of 2)than the Milky Way or M31.We note that,although possessing a Hubble type and bulge size similar to the Milky Way and M31,NGC 2683exhibits a disc size and HI gas mass that are significantly smaller.Rhode et al.(2007)show that the extent of the GC system of NGC 2683is also rather small,with the projected density of the sys-tem falling to background levels within ∼8kpc.This can be compared to the Milky Way GC system in which a fraction of the GC system lies outside ∼30kpc.Some properties of2Proctor,Forbes,Brodie&StraderMilky Way S(B)bc1–-20.91 2.53 5.05 4.05160±2010.70 M31Sb10.78-21.21 2.43 6.45 3.05400±551 1.32 NGC2683Sb27.2-20.3 2.54 1.720.62120±4060.90Globular Clusters in NGC26833 gc018:52:27.833:24:40.4S/N<1(Sky)–––––––––gc028:52:27.833:24:40.4Star–––147.496.320.9719.10 1.87 1.24 gc038:52:37.933:23:24.4–368(3)559(7)525(20)111.7-46.921.1219.52 1.60 1.92 gc048:52:35.333:24:31.4–602(16)552(1)590(20)87.323.522.8720.86 2.02 2.27 gco58:52:35.533:24:39.7Contam283(14)546(2)593(20)79.727.623.2821.39 1.89 2.66 gc068:52:38.233:24:07.5–491(3)563(2)530(20)78.6-19.022.1420.44 1.70 2.31 gc078:52:35.333:25:22.5–557(14)532(4)497(20)59.651.222.8321.33 1.51 2.86 gc088:52:35.633:25:35.5–560(4)396(5)437(20)66.239.321.2419.72 1.52 4.17 gc098:52:41.033:24:51.7–388(4)481(2)471(20)22.5-12.620.9518.98 1.97 2.96 gc108:52:43.533:24:16.8–317(15)447(31)477(20)25.0-59.422.8420.86 1.98 2.67 gc118:52:42.033:25:02.9–430(3)461(8)460(20) 5.7-13.521.3319.82 1.51 5.55 gc128:52:42.333:25:11.3Contam432(3)432(3)424(20)-2.9-10.221.8820.00 1.89 3.09 gc138:52:43.633:24:59.4S/N<10–500(27)–-6.0-30.222.7720.89 1.88 2.04 gc148:52:44.433:25:18.6–304(5)426(2)424(20)-26.6-23.721.2719.43 1.84 2.22 gc158:52:42.133:26:30.8Star–351(5)386(20)-57.347.820.8618.47 2.39 1.01 gc168:52:42.833:26:45.3–217(5)344(5)–-73.851.822.0519.97 2.08 2.96 gc178:52:43.133:26:54.1–435(5)385(7)–-82.755.421.5719.84 1.739.66 gc188:52:46.733:25:52.9–244(6)365(2)–-71.2-19.822.4820.99 1.49 2.18 gc198:52:47.733:25:54.1–352(4)371(4)304(20)-80.9-27.820.8519.20 1.65 1.89 gc208:52:48.033:26:26.1S/N<10247(21)295(2)237(20)-106.2-7.823.6121.62 1.99 1.77 gc218:52:50.433:25:46.9–711(5)259(5)329(20)-99.8-56.821.8920.50 1.40 2.52 gc228:52:49.033:26:30.9–307(49)307(2)329(20)-118.5-13.323.0121.54 1.47 2.67 gc238:52:50.033:26:45.9S/N<10456(43)285(4)–-137.9-11.523.3521.38 1.97 3.07 gc248:52:53.033:27:15.3Star–166(85)252(20)-185.2-17.322.0920.58 1.52 1.31Index Offset ErrorTable3.Index offsets required to match the Lick system(Section√3.2).Errors are taken as the error on the mean(i.e.rms/4Proctor,Forbes,Brodie &StraderFigure 1.A sample of GC spectra after broadening to the Lick resolution.The age sensitive Balmer lines are highlighted in grey.Metallicity sensitive features are marked by lines.The young GC (gc11;bottom)is compared to old GCs with similar colours (gc03and gc08).Candidate gc11has similar Balmer line-strengths,but significantly stronger metallicity-sensitive features than gc03and gc08,indicating a younger,more metal-rich stellarpopulation.Figure 2.The distribution of GC candidates with respect to the galaxy NGC 2683is shown.The red diamond marks the centre of the galaxy,while the line identifies the major axis.Red stars are candidates identified as being stars.Blue stars represent candi-dates contaminated by OB associations.Filled symbols are GCs for which stellar population analysis was performed.Open cir-cles are candidates whose signal-to-noise was too low for stellar population analysis.The open circle onthe extreme right is the candidate used for sky estimation (Section 2).Figure 3.ACS imaging showing three GC candidates (from top to bottom;gc05,gc11and gc12).Each image (B band;left and I band;right)is 10arcsec (∼360pc at 7.2Mpc)on a side.Candi-dates gc05and gc12are clearly projected against OB associations in NGC 2683itself,and contamination appears highly likely.On the other hand,there is no evidence for an OB association affect-ing the young GC candidate;gc11.The comparisons to SSP models were carried out us-ing the χ2-fitting procedure of Proctor &Sansom (2002)(see also Proctor et al.2004a,b and Proctor et al.2005)to measure the derived parameters;log(age),[Fe/H],[Z/H]and [E/Fe](a proxy for the ‘α’–abundance ratio;see Thomas et al.2003for details).Briefly,the technique for deriving these parameters involves the simultaneous comparison of as many observed indices as possible to models of single stellar pop-ulations (SSPs).The best fit is found by minimising the deviations between observations and models in terms of the observational errors,i.e.χ.We have shown this approach to be relatively robust with respect to many problems which are commonly experienced in the measurement of spectral indices and their errors.These include poor or no flux cal-ibration,poor sky subtraction and poor calibration to the Lick system.The method is similarly robust with respect toGlobular Clusters in NGC 26835many of the uncertainties in the SSP models used in inter-pretation of the measured indices;e.g.the second parameter effect in horizontal branch morphologies and the uncertain-ties associated with the Asymptotic-Giant Branch.It was shown in Proctor et al.(2004a)and Proctor et al.(2005)that the results derived using the χ2technique are,indeed,significantly more reliable than those based on only a few indices.The process by which the candidate spectra were com-pared to the models was iterative.First,fits were obtained for all the candidates using all the available indices.The pat-terns of deviations from the fits obtained was then used to identifyindividual indices that matched the models poorly (see Fig.4).These included the H δ,CN indices for which flux levels were generally too low for accurate determination and Mg 1and Mg 2indices which suffer from flux calibration sensitivity.These indices were excluded from the analysis and the fits performed again.These fits were carried out using a clipping procedure in which indices deviating from the model fit by more than 3σwere excluded,and the fit performed again.Many of these poorly fitting indices could be associated with known problems,e.g.the contamination of the Mgb index by the 5202˚A sky-line in low signal-to-noise candidates.Indices that are excluded on this basis are in parentheses in Table A1.On average,after all exclusions,10indices were used in each of the final fits.For each GC in the sample,errors in the derived param-eters (log(age),[Fe/H],[E/Fe]and [Z/H])were estimated us-ing 50Monte-Carlo realisations.Best-fit model indices were perturbed by Gaussians,the width of which were set equal to the observational errors added in quadrature to the er-rors in offset to the Lick system (Table 3).Error estimates in the derived parameters are therefore highly sensitive to the estimates of index errors.The process also makes no al-lowance for the correlated components of the observational index errors,such as velocity dispersion,flux calibration and background subtraction errors.The errors are modelled in-stead as purely random Gaussian distributions.As a conse-quence,our error estimates must be considered to include both random and systematic errors.4RESULTS4.1Results of recession velocity analysisThe results of our analysis of recession velocities are given in Table 2and are presented in Fig.5.The value assumed for the galactic centre (442.8km s −1)was estimated such that the value of the least-squares fit to the stellar RVs with radial distance (solid line in Fig.5)passes through 0.0km s −1at a radial distance of 0.0arcsec.Note that candidates identified as stars and the single object with signal-to-noise <1are omitted from these and all subsequent plots of GC data.We therefore present recession velocities for 19GCs.The stellar and gas emission-line data (Fig.5)clearly show an increasing rotation speed with increasing radial dis-tance from the galactic centre,and can be seen to be essen-tially cylindrical (i.e.there is little scatter and no particular trend in RV with distance above or below the least-square fit).The figure also shows the rotation of gas and stars to be in very good agreement.Figure 4.shown in terms of observational errors (i.e.χ).The open symbols identify the indices excluded from the fitting procedure.Error bars show rms scatter.Figure 5.Recession velocities are shown against radial distance from the galactic centre perpendicular to the minor axis.Solid black points are stellar data,while open symbols for the gas.The stellar and gas data clearly show rotation.The least-squares fit to the stellar data is shown as a line.The small scatter about the fit shows the rotation to be roughly cylindrical.Coloured points are the GC data with colour representing actual GC colour from Forde et al.(2007)for red (B-I >1.8)and blue (B-I <1.8)candidates.6Proctor,Forbes,Brodie&StraderIt is evident that we do not reach the radii at which rota-tion is observed toflatten.However,our results are neverthe-less consistent with the rotation of Casertano&van Gorkom (1991)and Broeils&van Woerden(1994),whofind similar rotation curves with aflattening/peak lieing just beyond the range probed by our data.The apparent dip in stellar and gaseous RVs at radial distance65arcsec in the rotation pro-file of Barbon&Capaccioli(1975)is also present at similar radii in our data(Fig.5;lower left),although we note that theyfind a significantly steeper rotation curve than Caser-tano&van Gorkom(1991),Broeils&van Woerden(1994) or ourselves.Our‘dip’is also significantly deeper than that observed by Casertano&van Gorkom,with both stars and gas rotating in the opposite sense to the rest of the galaxy at similar radii.The RVs measured in the GC spectra are also shown in Fig.5.GC recession velocities are generally consistent with those of the stars and gas.However,we lack sufficient numbers to unambiguously identify rotation in the GC system.4.2Results of stellar population analysisThe results of our age and metallicity determinations are given in Table4and plotted in Fig.6.Candidates with signal-to-noise<10are excluded from our analysis,leaving 15GCs suitable for stellar population analysis.In Fig.6our results are compared to the values for Galactic GCs from de Angeli et al.(2005)and Pritzl, Venn&Irwin(2005).It is shown in Mendel et al.(2007) that ages and metallicities derived from Lee&Worthey (2005)SSP models agree extremely well with Galactic GC measurements from colour-magnitude diagrams and high resolution spectral studies.For[Fe/H],Mendel et al.find only a0.028±0.024dex average offset between the value derived from Lee&Worthey SSPs models and the Harris(1996)values for42Galactic GCs.A similar offset (∼–0.024±0.021dex or–0.28±0.24Gyr)was found in the comparison of the derived ages with the data from de Angeli et al.(2005).Finally,the average values of[E/Fe] derived by Mendel et al.(2007)for Galactic GCs are offset from the Pritzl et al.(2005)values by–0.024±0.02dex(T. Mendel2007;private communication).The Mendel et al. (2007)results are consequently fully consistent with the literature data.We therefore have reasonable confidence in our comparison of the ages and metallicities of GCs of NGC2683with those of the Milky Way.4.3Consistency checkingHowever,before interpreting the ages and metallicity es-timates,we sought to gain further confidence in our re-sults by using them to predict the B-I colours of our GC sample(using the SSP models of Bruzual&Charlot2003) for comparison to the observed HST colours(Forde et al. 2007).The comparison is shown in Fig.7.The predictions compare quite favourably with the observed values,particu-larly given the∼0.15mag overestimation of predicted(B–I) colour found by Pierce et al.(2005,2006)in similar studies.This is believed to be primarily the effect of the poor mod-elling of the horizontal branch(see also Strader&Smith 2007).Scatter should also be expected to be relatively high in our study due to the highly variable internal extinction in NGC2683.There is,however,one clearly aberrant GC–gc11;an apparently young GC(Table4).We note that the spectrum of this GC is clearly different from other GCs of the same colour in a sense consistent with the derived younger age and higher metallicity,i.e.similar Balmer line strengths and stronger metal lines(see Fig.1).It is clear from Fig.7 that this effect is not the result of extinction.However,the proximity of this GC to the galactic centre makes contam-ination by the background galaxy a concern.We therefore experimented with adding galaxy light back into the GC spectrum and then subjecting the resultant spectrum to our age/metallicity analysis.We found that when50%of the galaxy light was recombined with the GC spectrum the derived age increased by0.2dex(i.e to5Gyr),while the metallicity fell by a similar amount,resulting in a similar predicted colour.This is both a relatively small change(for a relatively large amount of galaxy contamination)and is in the opposite sense to that required to explain the young age by galaxy contamination.We therefore conclude that background contamination in the spectroscopic analysis is unlikely to be the cause of the observed young age of this GC,or the discrepancy with its predicted colour.The cause of the discrepancy between observed and predicted colours therefore remains unknown.4.4Stellar population parametersHaving gained some confidence in our measured stellar pa-rameters we now return our attention to the age and metal-licity estimates.Our stellar population analysis identifies a single young GC,with a derived age of3.3Gyr.This is similar to the luminosity-weighted age of4.7Gyr found for the galactic centre by Proctor&Sansom(2002).The central[Fe/H]=–0.03±0.09and[E/Fe]=0.20±0.04found in Proctor&San-som(2002)are also similar to the values found for this young GC(–0.17±0.04and0.16±0.03respectively;Fig.6).This suggests the possibility that this GC formed in the same event that fuelled the central star-burst.Wefind the remaining14of15GCs to possess ages older than10Gyr(Fig.6).Infive cases wefind an age equal to the oldest age modelled by Lee&Worthey(2005). It is apparent that the scatter in GC age estimates is smaller than the error given by our Monte-Carlo analysis(Section 3.2).We take this to be a combination of three effects;i)the error includes both random and systematic errors,ii)the scatter is slightly suppressed by the GCs hitting the oldest age,iii)a slight over-estimation of observational errors is also a possibility(see Section3.2).The14GCs found to be old span a broad range of metallicities(Fig.6),similar to that observed in other spiral galaxy GC systems(Burstein et al.1984;Beasley et al.2004; Schroder et al.2002;Larsen et al.2002;Olsen et al.2004). They also span a similar range to the Milky Way GC system (de Angeli et al.2005;Fig.6).Fig.6also shows a comparison of[E/Fe]values fromGlobular Clusters in NGC26837 gc0311.9 1.08(0.15)-1.40(0.13)0.21(0.08)-1.20(0.16)gc0411.2 1.05(0.13)-0.99(0.17)0.09(0.08)-0.90(0.15)gc0611.9 1.08(0.20)-1.57(0.33)0.21(0.14)-1.38(0.24)gc0711.9 1.08(0.18)-1.63(0.26)0.24(0.11)-1.40(0.22)gc0810.0 1.00(0.08)-1.95(0.09)0.24(0.15)-1.73(0.13)gc0910.0 1.00(0.18)-0.72(0.17)0.05(0.07)-0.68(0.21)gc1010.0 1.00(0.18)0.16(0.18)-0.25(0.24)-0.08(0.24)gc11 3.30.53(0.02)-0.17(0.03)0.16(0.02)-0.03(0.03)gc1410.0 1.00(0.07)-0.53(0.12)0.01(0.11)-0.53(0.08)gc1611.2 1.05(0.07)-0.71(0.10)0.14(0.10)-0.58(0.12)gc1711.2 1.05(0.14)-1.47(0.12)0.15(0.05)-1.33(0.09)gc1810.0 1.00(0.10)-1.59(0.24)0.36(0.25)-1.25(0.16)gc1911.9 1.08(0.15)-1.26(0.11)0.12(0.05)-1.15(0.12)gc2111.2 1.05(0.04)-2.55(0.19)0.24(0.11)-2.33(0.18)gc2211.9 1.08(0.26)-2.05(0.56)0.27(0.27)-1.80(0.52)8Proctor,Forbes,Brodie&StraderFigure 6.Metallicity–age(top)andα-element abundance–metallicity(bottom)relations for NGC2683GCs.Values for theGalactic GC system are shown as small solid symbols.These arefrom de Angeli et al.(2005)(top)and Pritzl et al.(2005)(bot-tom).The relation for local Galactic stars is shown as a dashedline in the bottom plot for reference only.GCs in NGC2683with(B-I)0>1.8are shown as red squares,those with(B-I)0≤1.8asblue circles.Arrows indicate GCs whose derived age equals themaximum modelled by Lee&Worthey(2005).One GC(gc11;solid blue star)exhibits age,[Fe/H]and[E/Fe]similar to thethose measured in the galactic centre by Proctor&Sansom(2002)(open black stars).Combinedsystematic and random errors fromour Monte Carlo analysis(Section3.2)are indicated in each plot.6ACKNOWLEDGEMENTSWe thank Soeren Larsen for help preparing the slit maskand Kieran Forde for providing information prior to pub-lication.We also thank Lee Spitler for assistance with thephotometric analysis.Part of this research was funded byNSF grant AST-02-06139The data presented herein wereobtained at the W.M.Keck Observatory,which is operatedFigure7.A comparison between(B-I)values predicted fromBC03models using our derived ages and metallicities and the ob-served Galactic extinction corrected colours of Forde et al.(2007).Symbols as Fig.6.The solid line represents the one-to-one rela-tion.The extinction correction corresponding to A V=0.2is shownin the bottom right.Figure8.[Fe/H]is plotted against projected distance along themajor axis.Symbols as Fig.6.Lines represent the Harris(2000)trends for the average[Fe/H]with radius in Galactic GCs whensubdivided into red and blue subpopulations.as a scientific partnership among the California Institute ofTechnology,the University of California and the NationalAeronautics and Space Administration.The Observatorywas made possible by the generousfinancial support ofthe W.M.Keck Foundation.This research has made useof the NASA/IPAC Extragalactic Database(NED),whichis operated by the Jet Propulsion Laboratory,Caltech,under contract with the National Aeronautics and SpaceAdministration.We also thank the Australian ResearchGlobular Clusters in NGC26839Council for funding that supported this work.7REFERENCESArmandroffT.E.,Da Costa G.S.,Zinn R.,1992,AJ,104, 164Ashman K.M.,Zepf,S.E.,1992,ApJ,384,50Barbon R.,Capaccioli M.,1975,A&A,42,221Barmby P.,Huchra J.P.,Brodie J.P.,Forbes D.A.,Schroder L.L.,Grillmair C.J.2000,AJ,119,727Beasley M.A.,Brodie J.P.,Strader J.,Forbes D.A.,Proctor R.N.,Barmby P.,Huchra J.P.,2004,AJ,128,1623 Bedregal A.G.,Aragn-Salamanca A.,Merrifield M.R.,2006, MNRAS,373,1125Broeils A.H.,van Woerden H.,1994,A&AS,107,129 Bruzual A.G.,Charlot S.,2003,MNRAS,344,1000 Burstein D.,Faber S.M.,Gaskell C.M.,Krumm N.,1984, ApJ,287,586Casertano S.,van Gorkom J.H.,1991,AJ,101,1231 Chandar R.,Puzia T.H.,Sarajedini A.,Goudfrooij P.,2006, ApJ,646L,107Courteau S.,van den Bergh S.,1999,AJ,118,337De Angeli F.,Piotto G.,Cassisi S.,Busso G.,Recio-Blanco A.,Salaris M.,Aparicio A.,Rosenberg A.,2005,AJ,130, 116Eggen O.J.,Lynden-Bell D.,Sandage A.R.,1962,ApJ,136, 748Forbes D.A.,Beasley M.A.,Bekki K.,Brodie J.P.,Strader J.,2003,Science,301,1217Forbes D.A.,Brodie J.P.,Larsen S.S.,2001,ApJ,556,83 Forbes D.A.,Strader J.,Brodie J.P.,2004,AJ,127,3394 Forde et al.,2007,in preparationGilmore G.,King I.,van der Kruit P.,1989,Proceedings of the19th Advanced Course of the Swiss Society of Astron-omy and Astrophysics(SSAA),Saas-Fee,Leysin,Vaud, Switzerland,13-18March,1989,Geneva:Observatory, 1989,edited by Buser,Roland,p334Goudfrooij P.,Strader J.,Brenneman L.,Kissler-Patig M., Minniti D.,Huizinga J.E.,2003,MNRAS,343,665Harris W.E.,2000,“Star Clusters”,in“28th Saa-Fee Advanced Course for Astrophysics and Astronomy”Houdashelt M.L.,Trager S.C.,Worthey G.,Bell R.A.,2002, Elemental Abundances in Old Stars and Damped Lyman-Systems,25th meeting of the IAU,Joint Discussion15,22 July2003,Sydney,AustraliaIbata R.A.,Gilmore G.,Irwin M.J.,1995,MNRAS,277, 781Jensen J.B.,Tonry J.L.,Barris B.J.,Thompson R.I.,Liu M.C.,Rieke M.J.,Ajhar E.A.,Blakeslee J.P.,2003,ApJ, 583,712Kent S.M.,1985,ApJS,59,115Kissler-Patig M.,Ashman K.M.,Zepf S.E.,Freeman K.C., 1999,AJ,118,197Lanfranchi G.A.,Matteucci F.,2004,MNRAS,351,1338 Larsen S.S.,Forbes D.A.,Brodie J.P.,2001,MNRAS,327, 1116Larsen S.S.,Brodie J.P.,Beasley M.A.,Forbes D.A.,2002, AJ,124,828Lee H.,Worthey G.,2005,ApJS,160,176Lee Y-K.,Gim H.B.,Casetti-Dinescu D.I.,ApJ,661,L52Mackey A.D.,Gilmore G.F.,2004,MNRAS,355,504 Martin N.F.,Ibata R.A.,Bellazzini M.,Irwin M.J.,Lewis G.F.,Dehnen W.,2004,MNRAS,348,12Mendel J.T.,Proctor R.N.,Forbes D.A.,2007,MNRAS, 379,1618Merrifield M.R.,Kuijken K.,1999,A&A,345,47Oke J.B.,Cohen J.G.,Carr M.,Cromer J.,Dingizian A., Harris F.H.,Labrecque S.,Lucinio R.,Schaal W.,Epps H., Miller J.,1995,PASP,107,375Olsen K.A.G.,Miller B.W.,SuntzeffN.B.,Schommer R.A., Bright J.,2004,AJ,127,2674Pierce M.,Brodie J.P.,Forbes D.A.,Beasley M.A.,Proctor R.N.,Strader J.,2005,MNRAS,358.,419Pierce M.,Bridges T.,Forbes D.A.,Proctor R.N.,Beasley M.A.,Gebhardt K.,Faifer F.R.,Forte J.C.,Zepf S.E., Sharples R.,Hanes D.A.,2006,MNRAS,368.325Pritzl B.J.,Venn K.A.,Irwin M.,2005,AJ,130,2140 Proctor R.N.,Sansom A.E.,2002,MNRAS,333,517 Proctor R.N.,Forbes D.A.,Beasley M.A.,2004a,MNRAS, 355,1327Proctor R.N.,Forbes D.A.,Hau G.K.T.,Beasley M.A.,De Silva G.M.,Contreras R.,Terlevich A.I.,2004b,MNRAS, 349,1381Proctor R.N.,Forbes D.A.,Forestell A.,Gebhardt K.,2005, MNRAS,362,857Rhode K.L.,Zepf S.E.,Kundu A.,Larner A.N.,astro-ph/0708.1166Schroder L.L.,Brodie J.P.,Kissler-Patig M.,Huchra J,P., Phillips A.C.,2002,AJ,123,2473Searle L.,Zinn R.,1978,ApJ,225,357Strader J.,Smith G.,2007,ApJ,submittedThomas D.,Maraston C.,Bender R.,2003,MNRAS,339, 897Tonry J.L.,Dressler A.,Blakeslee J.P.,Ajhar E. A., Fletcher A.B.,Luppino G.A.,Metzger M.R.,Moore C.B., 2001,ApJ,546,681Trager S.C.,Worthey G.,Faber S.M.,Burstein D.,Gon-zarlez J.J.,1998,ApJS,116,1van den Bergh S.,1999,A&ARv,9,273Worthey G.,Ottaviani D.L.,1997,ApJS,111,377 APPENDIX A:LICK INDICES10Proctor,Forbes,Brodie&Stradergc030.613 2.496(-0.773) 1.357 2.3560.878 1.6670.856 2.407(3.319)(0.789) 1.459 1.3540.4730.1580.308(0.284)0.1500.3820.1950.2850.4130.202(0.396)(0.195)0.2300.2780.203gc040.826(2.314)-1.802(0.650) 4.043(2.170) 3.129 3.0860.849 3.717 1.704 1.537 1.434 1.0240.252(0.497)0.484(0.283)0.644(0.335)0.5130.7760.3470.7060.3270.3880.4570.341gc050.491 4.129-1.598 1.097(0.016) 1.410 2.178 3.120 2.710 5.260(3.361) 2.823 2.081(2.848)0.2970.5150.5430.316(0.800)0.4000.5960.8980.3750.847(0.406)0.4810.558(0.388)gc060.558 1.760 1.102 1.957(-0.892)0.026(4.640)-0.698(2.973)–(2.502)(2.594)(3.365)0.9750.2720.4980.4570.268(0.756)0.368(0.505)0.823(0.334)–(0.349)(0.401)(0.459)0.360gc070.674 1.421(2.184) 2.137 1.481(1.445) 1.969 1.189(1.944)(-0.967) 1.3590.712(2.443)(1.234)0.2590.493(0.455)0.2730.706(0.354)0.5670.853(0.357)(0.811)0.3670.445(0.508)(0.392)gc080.270(2.277) 1.095(2.398) 1.5250.543 1.005(-0.971) 2.817 1.963(0.889)0.527(-0.296)0.4780.139(0.277)0.245(0.121)0.3350.1700.249(0.354)0.1790.341(0.159)0.196(0.239)0.168gc090.987 4.497(-1.567)(1.870) 4.216 1.480 2.695(3.496)(3.054) 4.700 2.082 1.809 2.001 1.1390.1460.288(0.274)(0.138)0.3470.1760.261(0.375)(0.192)0.3740.1770.2130.2500.183gc10(2.085)(4.412)-6.486(-0.820) 5.984 1.888 3.711(-0.545)(3.132) 4.335(5.628) 3.803 3.382(-1.105)(0.266)(0.571)0.672(0.397)0.8060.4170.621(1.018)(0.378)0.908(0.402)0.4960.589(0.508)gc11(1.282) 4.757-3.7180.044 4.424 1.341 2.790 4.384 2.302(4.093) 3.153 2.577– 1.314(0.101)0.2260.1930.0810.2210.1100.1430.1600.136(0.192)0.0800.109–0.092gc14(2.066)(8.325)(-6.007)-0.872 3.526 1.497 2.655 2.910(2.760)(7.069)(6.047)(3.092) 2.487(2.594)(0.152)(0.310)(0.349)0.1980.4270.2190.3140.454(0.214)(0.407)(0.181)(0.232)0.278(0.193)gc16(1.335) 4.365-4.644-0.772 4.343 1.316 1.977(0.293) 1.886(5.444)(1.715) 2.616 1.721 1.147(0.177)0.3500.3640.2110.4540.2360.354(0.530)0.237(0.463)(0.227)0.2550.3110.226gc170.812 2.337-0.019(1.640) 1.692(0.951) 1.631(0.399)(2.379) 2.857 1.007(1.963)(1.882)0.6600.1340.2770.247(0.124)0.331(0.167)0.241(0.340)(0.177)0.3240.151(0.178)(0.220)0.159gc180.602(4.593)-1.0770.433 1.818 1.729(-0.295)(-3.244)(3.752)(6.285)(6.797) 1.817(2.002)0.3620.191(0.358)0.3790.2230.5530.259(0.452)(0.660)(0.273)(0.597)(0.260)0.364(0.429)0.340gc190.347 2.742-0.704 1.413 2.5080.893 2.543(1.323) 1.885 3.133 1.415(2.099) 1.6940.6570.1680.3190.2990.1600.4040.2080.307(0.454)0.2140.4260.205(0.241)0.2920.217gc210.4140.368 2.799 3.054 1.5600.6140.488(-3.468) 3.386 1.508(1.695)-1.085 1.1140.0110.2500.4700.4060.2280.6290.3260.533(0.810)0.3160.732(0.347)0.4510.5100.392gc220.554 1.470 2.364(3.414) 1.936 1.382-1.338––(-0.760)–(-2.791)(-3.424)(3.388)0.3500.7950.672(0.393) 1.0150.5700.967––(1.420)–(0.840)(1.076)(0.642)。
遥感与地理信息系统方面的好的期刊
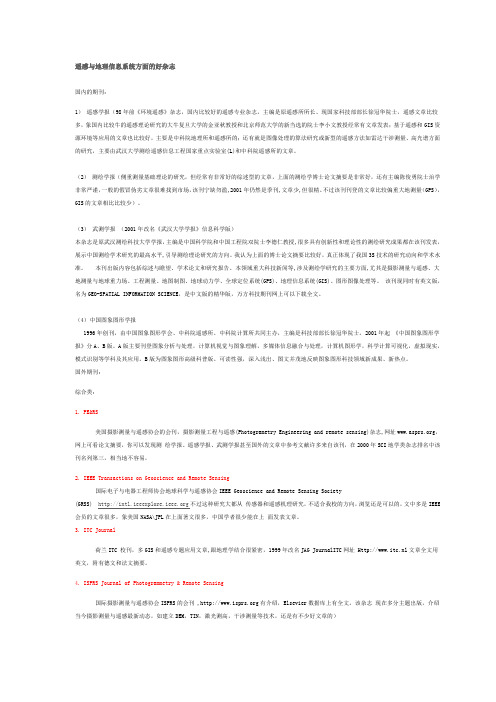
遥感与地理信息系统方面的好杂志国内的期刊:1)遥感学报(98年前《环境遥感》杂志,国内比较好的遥感专业杂志,主编是原遥感所所长、现国家科技部部长徐冠华院士,遥感文章比较多,象国内比较牛的遥感理论研究的大牛复旦大学的金亚秋教授和北京师范大学的新当选的院士李小文教授经常有文章发表;基于遥感和GIS资源环境等应用的文章也比较好,主要是中科院地理所和遥感所的;还有就是图像处理的算法研究或新型的遥感方法如雷达干涉测量、高光谱方面的研究,主要由武汉大学测绘遥感信息工程国家重点实验室(L)和中科院遥感所的文章。
(2)测绘学报(侧重测量基础理论的研究,但经常有非常好的综述型的文章,上面的测绘学博士论文摘要是非常好,还有主编陈俊勇院士治学非常严谨,一般的假冒伪劣文章很难找到市场,该刊宁缺勿滥,2001年仍然是季刊,文章少,但很精。
不过该刊刊登的文章比较偏重大地测量(GPS),GIS的文章相比比较少)。
(3)武测学报(2001年改名《武汉大学学报》信息科学版)本杂志是原武汉测绘科技大学学报,主编是中国科学院和中国工程院双院士李德仁教授,很多具有创新性和理论性的测绘研究成果都在该刊发表,展示中国测绘学术研究的最高水平,引导测绘理论研究的方向。
我认为上面的博士论文摘要比较好,真正体现了我国3S技术的研究动向和学术水准。
本刊出版内容包括综述与瞻望、学术论文和研究报告、本领域重大科技新闻等,涉及测绘学研究的主要方面,尤其是摄影测量与遥感、大地测量与地球重力场、工程测量、地图制图、地球动力学、全球定位系统(GPS)、地理信息系统(GIS)、图形图像处理等。
该刊现同时有英文版,名为GEO-SPATIAL INFORMATION SCIENCE,是中文版的精华版,万方科技期刊网上可以下载全文。
(4)中国图象图形学报1996年创刊,由中国图象图形学会、中科院遥感所、中科院计算所共同主办,主编是科技部部长徐冠华院士。
2001年起《中国图象图形学报》分A、B版。
Broad Band Dielectric Spectroscopy -williams
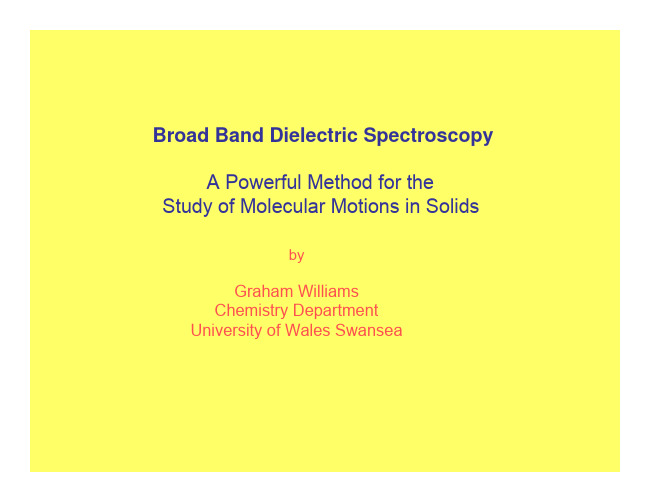
Snapshot of an ensemble at time t = 0 The local regions span the range of dynamical behaviour - giving slow to fast relaxations Motions occur in the local regions; continual exchange of energies between the regions changes their dynamics continually
ε (ω ) =
σ (ω ) 1 = M (ω ) iωε v
C0
Broadband Dielectric Spectroscopy
Complex Permittivity
ε = ε '−iε ' ' ε '= ε∞ +
∆ε 1 + ω 2τ 2
ε ''=
log f/Hz 1. Relaxation Strength ∆ε 3. Shape of process Low frequencies
Electrical Properties of Polymers
E Riande & R Diaz-Calleja, Marcel Dekker 2004
Introductory Review
G Williams & DK Thomas, Novocontrol Application Notes, No.3, 1998.
谱聚类算法(Spectral Clustering)原理分析
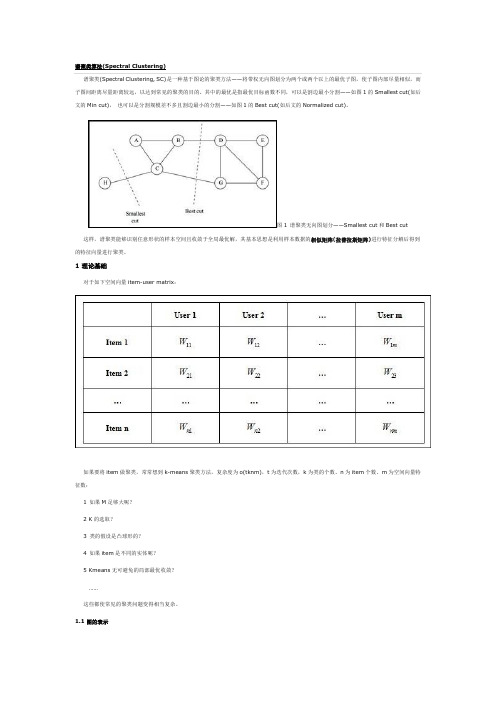
谱聚类算法(Spectral Clustering)谱聚类(Spectral Clustering, SC)是一种基于图论的聚类方法——将带权无向图划分为两个或两个以上的最优子图,使子图内部尽量相似,而子图间距离尽量距离较远,以达到常见的聚类的目的。
其中的最优是指最优目标函数不同,可以是割边最小分割——如图1的Smallest cut(如后文的Min cut),也可以是分割规模差不多且割边最小的分割——如图1的Best cut(如后文的Normalized cut)。
图1 谱聚类无向图划分——Smallest cut和Best cut 这样,谱聚类能够识别任意形状的样本空间且收敛于全局最优解,其基本思想是利用样本数据的相似矩阵(拉普拉斯矩阵)进行特征分解后得到的特征向量进行聚类。
1 理论基础对于如下空间向量item-user matrix:如果要将item做聚类,常常想到k-means聚类方法,复杂度为o(tknm),t为迭代次数,k为类的个数、n为item个数、m为空间向量特征数:1 如果M足够大呢?2 K的选取?3 类的假设是凸球形的?4 如果item是不同的实体呢?5 Kmeans无可避免的局部最优收敛?……这些都使常见的聚类问题变得相当复杂。
1.1 图的表示如果我们计算出item与item之间的相似度,便可以得到一个只有item的相似矩阵,进一步,将item看成了Graph(G)中Vertex(V),歌曲之间的相似度看成G中的Edge(E),这样便得到我们常见的图的概念。
对于图的表示(如图2),常用的有:邻接矩阵:E,e ij表示v i和v i的边的权值,E为对称矩阵,对角线上元素为0,如图2-2。
Laplacian矩阵:L = D – E,其中d i (行或列元素的和),如图2-3。
图2 图的表示1.2 特征值与L矩阵先考虑一种最优化图像分割方法,以二分为例,将图cut为S和T两部分,等价于如下损失函数cut(S, T),如公式1所示,即最小(砍掉的边的加权和)。
- 1、下载文档前请自行甄别文档内容的完整性,平台不提供额外的编辑、内容补充、找答案等附加服务。
- 2、"仅部分预览"的文档,不可在线预览部分如存在完整性等问题,可反馈申请退款(可完整预览的文档不适用该条件!)。
- 3、如文档侵犯您的权益,请联系客服反馈,我们会尽快为您处理(人工客服工作时间:9:00-18:30)。
a r X i v :a s t r o -p h /0010238v 1 12 O c t 2000K-Band Spectroscopy of Compact Star Clusters in NGC4038/4039Sabine Mengel,Matthew D.Lehnert,Niranjan Thatte,Lowell E.Tacconi-Garman andReinhard GenzelMax-Planck-Institut f¨u r extraterrestrische Physik,Postfach 1312,D-85741,Garching,Germanymengel,mlehnert,thatte,lowell,genzel@mpe.mpg.deABSTRACTIntegral field spectroscopy in the K-band (1.9-2.4µm)was performed on four IR-bright star clusters and the two nuclei in NGC 4038/4039(“The Antennae”).Two of the clusters are located in the overlap region of the two galaxies,and together comprise ≈25%of the total 15µm and ≈10%of the total 4.8GHz emission from this pair of merging galaxies.The other two clusters,each of them spatially resolved into two components,are located in the northern galaxy,one in the western and one in the eastern loop of blue paring our analysis of Br γ,CO band-heads,He I λ2.058µm,H α(from archival HST data),and V −K colors with stellar population synthesis models indicates that the clusters are extincted (A V ≈0.7−4.3mags)and young,displaying a significant age spread (4-13Myrs).The starbursts in the nuclei are much older (65Myrs),with the nucleus of NGC 4038displaying a region of recent star formation northward of its K-band ing our derived age estimates and assuming the parameters of the IMF (Salpeter slope,upper mass cut-offof 100M ⊙,Miller-Scalo between 1M ⊙and 0.1M ⊙),we find that the clusters have masses between 0.5and 5×106M ⊙.Subject headings:galaxies:individual:NGC 4038/4039,The Antennae —infrared:galaxies —galaxies:starburst —galaxies:interactions —stars:formation1.IntroductionArguably,one of the most fascinating recent as-trophysical discoveries is the rich population of young luminous blue “globular-like”clusters in strongly interacting and merging systems (Holtz-man et al.1992;Whitmore et al.1993,1997;Whitmore &Schweizer 1995,hereafter WS95).These clusters all appear much younger (<100Myrs)than both the old globular cluster sys-tem and underlying background population and have dynamical masses (M ∼few ×105M ⊙–in a few cases –Ho &Filippenko 1996)and effec-tive radii very similar to the globulars (r e ∼10pc –WS95).The formation of such clusters appears to be generic to the merger process and is likely the result of the high pressures that are induced dur-ing the merger process (e.g.,Elmegreen &Efremov 1997).The star formation activity in NGC 4038/4039–the “Antennae”–has been known from stud-ies at various wavelengths,for example from H αspectroscopy (Rubin et al.1970)or the very blue colors observed in the HST images (WS95).Its relative proximity (19Mpc assuming H 0=75km s −1Mpc −1)makes it the ideal candidate for the study of merger induced star formation on scales of single star clusters and has allowed for the detection and investigation of a very large num-ber (≈800)of compact clusters (Whitmore et al.1999,WS95).The heavily extincted overlap re-gion was revealed to be the most active star forma-tion zone by radio observations (Hummel &Van der Hulst 1986)and imaging and spectroscopy performed with ISO (Mirabel et al.1998;Kunze et al.1996;Fischer et al.1996).The over-lap region is,however,relatively inconspicuous at optical wavelengths.The ISO results suggestvery young ages for these clusters and the pres-ence of high mass(50-60M⊙)stars.Interest-ingly,a significant fraction of the total bolomet-ricflux from the Antennae appears to originate in two clusters eastward of the NGC4039nu-cleus(numbers86/87/88/89/90in WS95),build-ing up an optically bright knot,and number80in WS95,which is optically extincted and declared a“very red object”.These two regions,which are the highest surface brightness features in the mid-infrared,contribute≈25%of the totalflux at15µm(Mirabel et al.1998)and≈20%of the total emission from discrete radio sources in NGC4038/4039(Hummel&Van der Hulst1986). These large fractions suggest that these two re-gions must constitute a significant portion of the very recent or on-going star-formation within the Antennae.Understanding their properties within the perspective of the overall star formation his-tory of the Antennae is of prime importance to un-derstanding the bolometric output of these merg-ing galaxies.We discuss the properties of these re-gions as determined through IR integralfield spec-troscopy in combination with data available from the literature.2.Cluster Selection and ObservationsModels of the merger of two spiral galaxies(like that currently being observed in NGC4038/4039) predict multiple bursts of star formation during the merger event(Mihos and Hernquist1996). Depending on the stage of the merger,these bursts can be in the region of overlap of the two galaxy disks,in the tidally shocked regions of the outer disks of each galaxy,or as significant quantities of gas fall in towards the two nuclei,in the nu-clei of each galaxy.We observed the two most prominent clusters within the interaction zone, two other IR bright clusters in the outer arms of NGC4038/4039(likely to be tidally shocked re-gions),and the two nuclei.Such a selection would allow us(at a minimum)to probe the various regions of star-formation during the merger pro-cess within the inevitable constraint of only hav-ing a limited amount of observing time.While only observing six regions will not allow us to investigate the properties of the whole ensemble of compact clusters in the Antennae,our sam-ple allows us to crudely investigate the possi-ble range of ages across the Antennae.Figure 1shows the two clusters in the interaction zone ([WS95]86and[WS95]80),the two nuclei(NGC 4038and NGC4039)and two clusters in the outer loops([WS95]417/[WS95]428in the eastern and [WS95]405in the western),observed with the3D integralfield spectrometer(Weitzel et al.1996; Krabbe et al.1995)in the K band.For the observations,3D was combined with the tip-tilt-guider ROGUE(Thatte et al.1995)on the Anglo-Australian Telescope in February,1998 and April,1999.We used a K-band grism with a resolution ofλ/∆λ=1000and a256x256NIC-MOS3array.256spectra are obtained simultane-ously,arranged in a16x16pixelfield on the sky. The detector integration time per exposure was 100seconds.The on-source exposures were inter-leaved with off-source(offset60′′E of each cluster) exposures of identical integration time for the sky subtraction.To remove telluric features,the A2V star HD106819was observed roughly once every hour.In order to Nyquist sample the spectra,we dithered in the spectral direction by half a pixel using a piezo-driven mirror.The spatial pixel scale of0.′′4provided afield of view of6.′′4x6.′′4.The conditions were generally not photomet-ric andflux calibration achieved via aperture pho-tometry available from the literature(Bushouse &Stanford1992)in combination with Ks-band imaging(see also next section).The seeing dur-ing the observations of each individual region only showed moderate variation(<0.′′2)but was signif-icantly different for different objects.The values for each observation are discussed in section4. The total on-source integration time was6200s on thefirstfield(hereafter abbreviated to[WS95]86), which is the optically bright star cluster,cor-responding to the numbers86/87/88/89/90in WS95;4200s on-source time for the secondfield (hereafter[WS95]80),which corresponds to the “very red object”number80in WS95;3120s on-source time for thefield in the eastern loop(num-bers417and428in WS95);3840s for the targets in the western loop(WS95-number405and sev-eral fainter objects,hereafter[WS95]405).The nuclei of NGC4038and NGC4039were observed for3360s and2160s respectively.Due to a piezo failure,the spectrum of the nucleus of NGC4039 is not Nyquist sampled.3.Data Reduction and AnalysisData reduction was performed using the3D data reduction routines developed at MPE within the GIPSY(van der Hulst et al.1992)data reduction environment.Single frames were dark subtracted,linearized and sky subtracted using the sky frame that was closest chronologically and had the right spectral dithering position.The wavelength calibration was accomplished by ex-posures of a neon discharge lamp.The re-binning of the spectrally interleaved data was performed onto a600pixel linear wavelength axis.Bad pixels and cosmic rays were removed from the 16x16x600data cube,either by interpolation or by masking out.After spatial re-binning onto a 64x64pixel grid,the single cubes were stacked by centering each on its K-band continuum peak or the Brγpeak for[WS95]86and[WS95]80,respec-tively.Telluric features were removed by division by the atmospheric transmission profile obtained from the observations of the A2V star.The Brγabsorption line in the calibrator spectrum was in-terpolated linearly between the adjacent contin-uum.In the resulting cluster spectra,only the NGC4038nucleus spectrum shows a little remain-ing Brγemission from the calibrator,which is not a worry,because it is at zero redshift and does not contaminate the Brγemission from the object.Object spectra were extracted using a square aperture centered on the continuum peak and sized to maximize the SNR of the resulting spec-trum.The individual apertures and their sizes are shown in Figure2.Forfields which showed signifi-cant offsets between continuum and Brγline emis-sion peaks([WS95]405and the nucleus of NGC 4038),the aperture sizes were chosen so as to min-imize contamination between the continuum and line emitting regions.The spectra wereflux cali-brated using the K s-magnitudes from ourflux cal-ibrated SOFI image,obtained in May99at the NTT.The extracted spectra were normalized by dividing by the continuum value,estimated using a linearfit.From this we estimated the equivalent widths Wλof the Brγ-and the He I-lines and the CO-band-heads.For the creation of linemaps,the linearfit was performed for each spatial pixel and subtracted.The spectra of all the regions(divided into subregions for some of thefields)are shown in Fig.2,together with the K s-band images.Over-laid are the contours of Brγor the H2(1-0)S(1)-line (labelled in Fig.2).4.ResultsResults obtained from our data,incorporating results from publicly available HST data(Hαand V-band images–Whitmore et al.1999),are listed in Table1.[WS95]86was slightly resolved while [WS95]80was unresolved(both combined data sets had afinal FWHM∼1.′′0;Fig.2).The FWHM of the seeing was∼0.′′8during[WS95]417&428ob-servations,and the two clusters are marginally re-solved into single components.During[WS95]405 observations,the seeing was poor(1.′′6),but to the NE of the bright cluster,a fainter second cluster is visible.The tabulated values for[WS95]405-A and B refer to the bright cluster(A)and to the location of the Brγpeak(B).We used the measured equivalent width of Brγ, W Brγ,and CO2.29µm band-head,W CO(Table 1),to determine the ages of the starbursts using stellar synthesis models(Leitherer et al.1999; Kovo,Sternberg&Alexander1998).See plots in Leitherer et al.(1999)(their Figures89and 101),showing the evolution of these parameters with burst age.Assuming an upper mass cut-offM upper=100M⊙,Salpeter IMF slope and an instantaneous burst at solar metallicity,we de-rive ages of 3.7+1.0−0.4×106yr for[WS95]86and5.5+0.7−0.8×106yr for[WS95]80.Gilbert et al.(2000) derive a value of∼4Myrs for[WS95]80,assum-ing the same starburst parameters,in reasonable agreement with our value.The ages of the two clusters in[WS95]417&428are not very well con-strained,due to the low S/N of the data and the ambiguous value of the W CO.However,the values of W CO are sufficiently large to exclude additional errors introduced by the non-inclusion of the ther-mally pulsing asymptotic giant branch phase in the Geneva stellar evolution tracks that were in-corporated in Leitherer et al.(1999)(Origlia& Oliva2000).Age estimates of[WS95]417&428lie between8.5and12.8Myrs old.For the[WS95]405 spectra,it is not possible to get a consistent model age with the presence of both strong CO-band-heads and Brγemission with an instanta-neous burst.But given the size of the cluster (R eff=12.3pc,Whitmore et al.1999)it is plau-sible to assume that the star-formation has afi-nite duration of a few Myrs(Efremov&Elmegreen 1998).Other possibilities that might explain why these clusters have both CO absorption and Brγemission are shock heating of the gas due to super-nova explosions,the seeing broadened Brγemis-sion from the region to the S-E of[WS95]405-A which contaminates the cluster spectrum,superso-lar metallicity,and possible gaps in our knowledge of stellar evolution and model atmospheres.It is interesting to compare the3D image and spectra of a region outside both[WS95]405clusters with the multi-color HST image,since our data show a Brγpeak to the SE of the K-band continuum peak of cluster A.This coincides with a dustfila-ment obvious in the HST image,and it could be the site of more recent star formation or of shock excitation in a more dusty environment.A detailed discussion of the nuclear spectra is beyond the scope of the current paper.Here we only wish to emphasize how the spectra of the two nuclei differ from the young compact clusters.The W CO of the two nuclei suggest the same possi-ble age range for both,65±15Myrs,considerably older than the ages estimated for the prominent young clusters.The northern nucleus also shows evidence for a more recent starburst to the north of the K-band peak,but it is difficult to estimate its age due to the underlying continuum contribu-tion by the older background nuclear stellar pop-ulation.But the presence of Brγemission(no evi-dence for AGN activity was seen in other observa-tions so far)requires the presence of O orB stars or recent shock excitation,thus implying that it is younger than∼10-20Myrs.Under the assumption that there is no intrinsic gradient in W CO across the NGC4038nucleus,meaning that the observed lower W CO at the location of the Brγpeak can be attributed to the additional continuum from the young stellar population,we estimate the young star light continuum to contribute about1/5of the total continuum,which increases the observed W Brγto58˚A.This then implies an age of the star-burst of around6Myrs,consistent with the effec-tive temperature of33000K derived from the low ratio of He I/Brγof0.17.The age uncertainties given in Table1only take into account uncertainties in the signal-to-noise of the data and do not take into account the system-atic effects of selecting different IMF slopes,upper and lower mass cutoffs,abundances,or uncertain-ties in the models themselves.The extinction was derived by comparing the theoretical ratio of Hα/Brγ(case B recombina-tion ratio assuming T e=10000K from Storey& Hummer1995)with the observed ing the extinction curve of Draine(1989)for a fore-ground screen model,we derived A V of1.4±0.2 and4.3±0.2for[WS95]86and[WS95]80,respec-tively.They agree within the errors with the val-ues we derived from the V-K color excess with the intrinsic color derived from the models for the re-spective cluster ages(A V=1.6+0.6−1.0and3.7+0.6−1.0). For[WS95]417&428and[WS95]405,we used the V-K colors not to confirm the extinction,but rather to further constrain the age.The ratio of He Iλ2.058µm/Brγis not an un-ambiguous tracer of the effective temperature of the highest mass stars present in a stellar popula-tion(Shields1993;F¨o rster-Schreiber1998),but it was determined to compare with the ISO results at higher spatial resolution.The effective temper-atures derived from the ratios of He I/Brγ=0.47 and0.5are36,000and38,000K([WS95]86and [WS95]80,respectively),which corresponds to masses of roughly28M⊙and is below the44,000K (50-60M⊙)derived from mid-infrared line ratios by Kunze et al.(1996).Given the sensitivity of the He Iλ2.058µm line to nebular parameters (Shields1993)and the large aperture(14”×27”) used for the ISO measurement,some disagreement between our estimate and that obtained using ISO data for the temperature of the hottest stars is not surprising.Moreover,the relationship between temperature of the hottest stars and He I/Brγis not monotonic and thus our estimates for the mass should be considered lower limits.These up-per mass limits are consistent with our estimated ages of the[WS95]80cluster,while the younger age of[WS95]86would allow for the detection of higher mass stars.A qualitative comparison of the derived effective temperatures/ages of all fields agrees with the gradient in the[Ne III]/[Ne II]observed by Vigroux et al.(1996).From the extinction corrected Brγflux we can estimate the number of Lyman continuum photons,N Lyc.For this calculation,we used the extinction corrected Brγflux,a distance of19.2Mpc for the Antennae,and recombina-tion coefficients from Hummer&Storey(1987) and Storey&Hummer(1995).Wefind thatN Lyc=3.1±0.3×1052s−1and 1.9±0.4×1052s−1 for[WS95]86and[WS95]80respectively.This somewhat lower than the value derived from the thermal radio continuumflux density estimated by Hummel&Van der Hulst(1986),which re-sults in N Lyc=1.3±0.7×1053s−1for the sum of[WS95]86and[WS95]80.Given that our aper-tures are smaller,the difficulty in estimating the thermal contribution to the total radio emission, and that there is some diffuse radio emission,it is not surprising that there is a difference be-tween the two estimates.It is also significantly less than the value of1.0×1053photons s−1de-rived by Gilbert et al.(2000).Both our observed flux and estimated extinction are lower by factors of∼2and both of these differences account for the discrepancy between our respective estimates of the Lyman continuumflux.However,Gilbert et al.(2000)caution that the extinction value they derived(A K=1.1mag)should be considered an upper limit.Comparing our results(M K(0)and N Lyc) with the models of Leitherer et al.(1999,as-suming a Salpeter IMF slope,M upper=100M⊙, high mass stars have already evolved offthe main sequence and are therefore not observed,and M lower=1M⊙)for the age determined for each cluster in the previous section,the total mass ofeach cluster would be 1.6+1.2−0.2and 3.0+3.6−0.7×106M⊙for[WS95]86and[WS95]80respectively.A lower mass limit equal to0.1M⊙(using Miller-Scalo slope for the lower mass end of the IMF) would imply masses a factor of1.6higher.For [WS95]417&428and[WS95]405,we crudely esti-mated their masses using only their extinction-corrected K-magnitudes compared to the mag-nitudes expected from our derived ages.Their masses are lower than the masses of[WS95]86 and[WS95]80,and lie between0.3and1.1×106 M⊙.This places each of the very massive[WS95]86 and[WS95]80clusters into the same mass regime as M82A,which produces roughly the same amount of N Lyc,and has the same burst age (F¨o rster-Schreiber1998).Their masses are more than a factor of ten higher than the average mass of a globular cluster(Mandushev et al.1991). Even if they are expected to lose60%of their mass over a Hubble time(Chernoff&Weinberg1990), they will still represent the top-end of the globular cluster mass function.The[WS95]417&428and [WS95]405clusters have masses which are more moderate and comparable to the average globu-lar cluster mass.This mass range in the observed clusters is not surprising given that those twofields contribute a substantial amount of the bolometric luminosity of the Antennae.But a more accurate mass estimate of a larger fraction of the popula-tion of young star clusters is necessary to judge if the young clusters could evolve to form a part of the globular cluster population of an elliptical galaxy.5.Summary and ConclusionsWe performed integralfield spectroscopy in the K-band(1.9-2.4µm)on four star clusters and the nuclei in NGC4038/4039in order to derive the starburst properties and their variations with the location in the merger.Our analysis of Brγ,CO band-heads,He Iλ2.058µm/Brγ,Hα,and V-K colors indicates that the clusters show a consider-able age spread from4-13Myrs.The two star clusters in the interaction zone have ages near the lower limit of that range,while those in the outer loops lie near the top of that age range. These clusters sample the overall age gradient vis-ible in the Antennae,the details of which we will discuss in a forthcoming paper.The equivalent widths of the CO band-heads indicate that the starbursts in the nuclei are considerably older(65 Myrs;with the nucleus of NGC4038having evi-dence for a significant contribution from younger population with an age of∼6Myrs northward of its K-band continuum peak).The extinction is highly variable and especially high in some parts of the overlap region(A V=0.2to4.3).Using these age estimates and assuming the parameters of the IMF(Salpeter slope,upper and lower mass cut-offs,etc.),wefind that the clusters have masses ranging between0.3to3×106M⊙,(larger if the IMF is extended below1M⊙).Our observations that the K-band continuum peak(with its strong CO absorption)and the highest surface bright-ness Brγemission are not coincident in some re-gions and possible variations in W CO for clusters in[WS95]405and the nucleus of NGC4038argues for sequential star formation on small scales.We thank the staffof the AAT for their excel-lent assistance at the telescope and the editor S. Willner and the referee for helping improve the presentation of our paper.REFERENCESBushouse H.A.&Stanford S.A.,1992,ApJS,79, 213ChernoffD.F.,Weinberg M.D.,1990,ApJ,351, 121Draine,B.T.1989,in Proc.of the22nd ESLAB Symposium on Infrared Spectroscopy in As-tronomy,ed.B.H.Kaldeich(ESA SP-290),P.93Efremov,Y.&Elmegreen,B.1998,MNRAS,299, 588Elmegreen,B.&Efremov,Y.1997,ApJ,480,235Fischer,J.et al.1996,A&A,315,97F¨o rster-Schreiber,N.M.1998,Ph.D.thesis,der Ludwig-Maximilians-Universit¨a t M¨u nchenGilbert, A.M.et al.2000,preprint(astro-ph 9912369)Ho,L.&Filippenko,A.1996,ApJ,472,600Holtzman,J.A.et al.1992,AJ,103,691Hummel,E.&Van der Hulst,J.M.1986,A&A, 155,151Hummer,D.G.&Storey,P.J.1987,MNRAS, 224,801Kovo,O.,Sternberg.A.,&Alexander,T.1998, private communicationKrabbe,A.,et al.1995,ApJ,447,95Kunze,D.et al.1996,A&A,315,101Leitherer,C.,et al.1999,ApJS,123,3Mandushev G.,Spassova N.&Staneva A.,1991, A&A,252,94Mihos J.C.,Hernuist L.,ApJ,464,641Mirabel,I.F.,et al.1998,A&A,333,1Oliva E.,Origlia L.,Kotilainen J.K.,MoorwoodA.F.M.,1995,A&A,301,55Origlia L.&Oliva E.,astro-ph0003131Rubin V.et al.1970,ApJ,160,801Shields J.1993,ApJ,419,181Storey,P.J.&Hummer,D.G.1995,272,41Thatte,N.A.,Kroker,H.,Weitzel,L.,Tacconi-Garman,L.E.,Tecza,M.,Krabbe,A.,&Gen-zel,R.1995,SPIE,247,228.van der Hulst,J.M.,Terlouw,J.P.,Begeman, K.,Zwister,W.,&Roelfsema,in Astrophysi-cal Data Analysis Software and Systems I,(eds.D.M.Worall,C.Biemesderfer,and J.Barnes),ASP Conf.series num.25,p.131Vigroux,L.et al.1996,A&A,315,L93Weitzel,L.,et al.1996,A&AS,119,531Whitmore, B. C.,Schweizer, F.Leitherer, C., Borne,K.,&Robert,C.1993,AJ,106,1354 Whitmore,B.&Schweizer,F.1995,AJ,109,960 Whitmore,B.C.,Miller,B.W.,Schweizer,F.,& Fall,S.M.1997,AJ,114,2381Whitmore,B.C.,Zhang,Q.,Leitherer,C.Fall,S.M.,Schweizer,F.,&Miller,B.W.1999,AJ, 118,1551Fig. 1.—The HST V-band image(Whitmore et al.1999)displayed in a logarithmic grayscale with the 3D-fields and their numbering(referring to Whitmore&Schweizer(1995))as used in this paper indicated. The overall morphology is that of two colliding gas-rich spiral galaxies(likely Sc type).Eastward of the line connecting the two nuclei lies the so-called“interaction zone”or“overlap region”,which is heavily extincted in the optical.The size scale is indicated in the image.Fig. 2.—K-band images of the observedfields with Brγor H2contours,K-band spectra and the corre-sponding regions in color composite images taken from W99(blue:U+B,green:V,red:Hα).The greyscale images in the left column(top)are created from the3D data cubes by summation of the corresponding channels of the data cubes,continuum subtracted in the case of the emission line maps.They show the Brγdistribution in[WS95]86,[WS95]80,[WS95]417&428,[WS95]405and nucleus of NGC4038,and the H2(1-0)S(1)distribution in the nucleus of NGC4039.The highest contours represent95%of the maximumflux, then from90%continuing in steps of10%downward.Maximumfluxes are2.0([WS95]86),1.7([WS95]80), 0.47([WS95]405),0.59(NGC4039nucleus)and0.46(NGC4038nucleus)×10−16erg s−1cm−2.The K magnitudes of thefields are given in Table1.The spectra were extracted from the regions indicated in the K-band images.Thefluxes are given in units of10−16erg s−1cm−2versus the wavelength inµm.The noise level is indicated in the top right corner of each spectrum.The images in the left column(bottom)show the corresponding regions of the HST multi-color image(W99)with U+B in the blue,V in the red and Hαin the red display.The scale is given as1′′bars(the pixel size in all3D images is0.′′2x0.′′2).At the distance of the Antennae1′′corresponds to93pc.N is up,E to the left in each image.Table1Observed Properties of the Six FieldsProperty Unit[WS95]86[WS95]80[WS95]417[WS95]405-A NGC4039NGC4038[WS95]428[WS95]405-B nucleus A and B(1)(2)(3)(4)(5)(6)(7)(8)Note.—Col.(1)—Measured properties with1σuncertainties.Apert.size is the size of the apertures from which the values were derived.W Brγindicates the equivalent width of the Brγline,W CO is the equivalent width of the12CO (2-0)bandhead feature,determined in the wavelength range2.3060-2.3092µm,which corresponds to the wavelength range given by Oliva et al.(1995),redshifted to the radial velocity of the Antennae.Its uncertainty was estimated from the neighbouring continuum.For comparison,a few values of W CO for stars observed with3D:K4.5Ib:15.3˚A, M3.5Iab:19.6˚A,K3III:9.1˚A,M1III:12.1˚A(F¨o rster-Schreiber1998).Age is the model age(instantaneous burst, Salpeter IMF between1and100M⊙,solar metallicity)that most closely matches the equivalent widths of Brγand CO. Where the value for W CO covered a range that did not behave monotonically with age,we gave an age range rather than a value±σ.T eff of the most massive stars as indicated by the He I/Brγratio,He I/Brγmeasured from the3D spectroscopy,m K from our spectroscopy,m V from HST images(Whitmore et al.1999),A V from theflux ratio of Brγ/Hα,F Brγas measured from our data,F Hαfrom HST images(Whitmore et al.1999).Col.(2)—Unit in which the measurements in Cols.(3)to(8)are tabulated.Col.(3)to(8)—The measured properties(given in Col.(1))of the six observedfields.The values for the properties of NGC4038-B(Age,T eff and the value for W Brγin parentheses) make use of an assumption about the continuum level,see text for details.Moreover,the age determination of the nuclei may be influenced by the non-inclusion of the TP-AGB phase in the Starburst99models.。