BUS AND RING SYSTEM INTERCONNECTIONS FOR DATA ACQUISITION AND CONTROL
计算机网络与互联网(英语)chap8
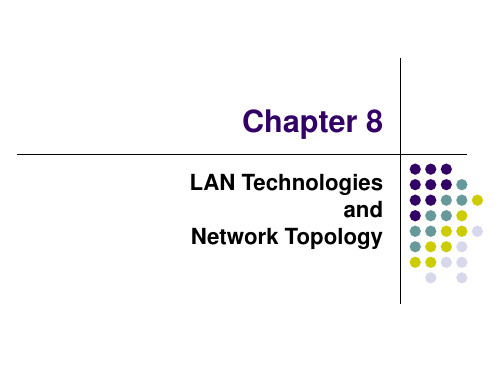
Disadvantages
Requires more cable length than a linear topology.
If the hub or concentrator fails, nodes attached are disabled.
The number of connections passing between two locations can exceed the total number of computers being connected
Direct Point to Point Calculating Required Lines
Classification Terminology
Network technologies classified into three broad categories
Local Area Network (LAN) Metropolitan Area Network (MAN) Wide Area Network (WAN)
1000mbps1gbpssharedmediuminalan?sharedmediumusedforalltransmissions?onlyonestationtransmitsatanytime?stationstaketurnsusingmediummediaaccesscontrolmacpolicyensuresfairness?mediaaccesscontrolmacpolicyillustrationofethernettransmissionccddaaeebbcomputerdreceiveacopyofeachbit?onlyonestationtransmitsatanytime?signalpropagatesacrossentirecable?allstationsreceivetransmission?csmacdmediaaccessschemesendingcomputertransmitsdatafrombtodrefuserefuserefuseacceptcsmacdparadigm?ethernetemployscsmatocoordinatetransmissionamongmultipleattachedcomputers?nocentralcontrolmanagingwhencomputerstransmitonether?multipleaccessma?multiplecomputersattachtosharedmedia?eachusessameaccessalgorithm?carriersensecs?waituntilmediumidle?begintotransmitframe?simultaneoustransmissionpossiblecsmacdparadigmcontinued?evenwithcsmatwocomputersmaytransmitsimultaneously?bothchecketheratsametimefinditidleandbegintransmitting?windowfortransmissiondependsonspeedofpropagationinether?signalsfromtwocomputerswillinterferewitheachothersignalsfromtwocomputers
专业英语词组

Generation发电delivery 输电consumption 消费electric power system电力系统power system电力系统power generation电能生产power transmission system输电系统power distribution systems配电系统power plant发电厂power station发电厂electric energy电能Transmission systems配电系统generation plants 电厂distribution systems 配电系统power systems 电力系统interconnections互联distribution system 配电系统individual loads 个体负荷transmission lines 输电线substations 变电站voltage transformation 变压器switching functions开关功能subtransmission中压输电neighboring power systems附近电力系统generating sources 发电原料transmission networks输电网络structural redundancy 结构冗余withstand unusual contingencies 承受意外事故service disruption 供电中断the radial system放射系统the loop system 环状系统the network system网状系统Distribution circuits 配电电路high-voltage transmission lines 高压输电线line sections线路截面direct current (DC) 直流alternating current (AC) 交流Industrial loads 工业负荷residential and 居民负荷commercial loads 商业负荷subsystems子系统synchronous 同步的synchronize 同步,使同步principle 主要的,首要的turbine 涡轮机,汽轮机prime mover 原动机,原动力bus 母线,总线frequency 频率in phase 同相地phase sequence 相序hydropower 水电hydropower plants 水电厂,水电站hydroelectric 水电的,水力发电的hydraulic 水力的,水压的couple 连接,耦合recreational 休养的,娱乐的fuel v. 得到燃料,刺激,推动n. 燃料,动力dissolved 溶解的,溶化的oxygen 氧riverbank 河堤,河岸downstream 下游的installation 装置,设备connotation 储蓄的东西thermal 热的,热量的combustion 燃烧化石燃料fossil fuelrating 额定condense 凝结,冷凝steam-turbine (蒸)汽轮机escalation 扩大,不断增加reconversion 恢复原状,再转变gas turbine 燃气涡轮机minor extent 小幅度peak load 最大负荷,峰荷nuclear station 核电站reaction 反应,反作用,感应fission 裂变cooling water 冷却塔uranium 铀fast breeder reactor 快中子增殖反应堆nuclear fusion 核聚变feasible 可行的petroleum 石油synthesize 综合,合成backbone 中枢integrated 综合的,完整的voltage level 电压等级step up 升压step down 降压subtransmission 中压输电,次输电subsystem 子系统bulk 大量的demarcation 划分relegate to 委托给,移交给overhead line 架空线cable 电缆megavoltampere MV Athermal limits 发热限制voltage drop 电压降落synchronism 同步性optimum 最优的voltage class 电压等级annealing 退火mechanical strength 机械强度sag 下陷,暂降clearance 间隙,距离statutory minimum 法定最小限度content 比例ampacity 安培容量,载流容量ambient 周围的solar radiation 太阳辐射bundle 捆,束,包neutral 中性点lightning shielding 避雷resistance 电阻inductance 电感capacitance 电容conductance 电导leakage 泄漏insulator 绝缘体impedance 阻抗shunt 旁路,并联admittance 导纳equivalent circuit 等效电路lumped parameter 集中参数uniform distribution 均匀分布non-uniformity 不均匀pronounced 显著的,明确的skin effect 集肤效应oscillation 振荡equilaterally 等边地flux linkage 磁链1电压等级变化transformation of voltage levelstep up升压step down降压2. 输电系统transmission system transmission subsystem 输电子系统subtransmission system中压输电系统3. 变电站substationtransmission substation输电变电站distribution substation配电变电站输电线transmission linetransmission line输电线,传输线overhead line 架空线power line电力线cable电缆series-in series with串联shunt-in parallel with 并联,旁路high energy consuming 高耗能的metallurgical 冶金的settlement center 结算中心intact 完整无缺的agricultural irrigation 农业灌溉compressor 压缩机refrigerator 电冰箱oven 烤箱,烤炉deep fryer 煎锅incandescent light 白炽灯fluorescent light 荧光灯converter 转炉;变流器attributable 可归于……的discharge lamp 放电管mercury 水银,汞vapour 蒸气sodium 钠electromagnetically 电磁控制地contactor 电流接触器dropout 退出trip 跳闸thermostatic 温度调节控制的sustained 持续的capacitor bank 电容器组explicitly 明确地implicitly 含蓄地trip 跳闸block 闭锁plunger 活塞balance-beam 平衡木robust 坚固的,耐用的immune to 免受……的harsh 残酷的solid-state 使用电晶体的,不用真空管的analog circuit 模拟电路pilot 引导,引航gear 设备,传动装置self-explanatory 不需加以说明的maloperation 误操作dielectric 电介质,绝缘体deenergize 去激励,断开conceivable 可想象的overlap 重叠1. 机电继电器electromechanical relays plunger type relay 插棒式继电器balance-beam type relay平衡杆式继电器rotating cup relay 转盘式继电器disc relay圆盘式继电器2. 继电器种类relay classesmagnitude relay幅度继电器directional relay定向继电器,方向继电器ratio relay比率继电器differential relay差动继电器pilot relay控制[引示、辅助]继电器3. 保护与后备protection and backup main protection主保护primary protection主保护backup protection 后备保护local backup protection本地后备保护breaker-failure backup protection 断路器失效后备保护remote backup protection远后备保护unit protection 组合保护busbar 母线ever-increasing 渐增的,不断增长的pilot wire 导引线invariably 不变的,总是e. m.f. (electromotive force)电动势fraction 小部分,片断interim 中间的,临时的negative-sequence 负序delta 三角形inrush 涌入conservator 储油器hinged 装铰链的float 漂浮物bubble 泡沫,气泡chamber 腔,室band 波段,频带intertrip 联锁跳闸modulation 调制bandpass filter 带通滤波器switchgear 开关设备short circuits 短路open circuits开路stability margins 稳定裕度heavy currents过载电流insulating oil 绝缘油cascading级联earth fault 接地故障clearance times 故障切除时间shock exposure time触电时间shielded double circuit 屏蔽双电路Super Grid 超级电网wood pole single circuits 木杆单回路extended earthing system扩展接地系统underground cables地下电缆earth impedance 对地阻抗shielding wire 屏蔽线mutual coupling 互耦合phase conductor相导体earth wire 地线lead sheaths 铅外套insulating layer 绝缘层Overhead lines架空线for the most part在极大程度上,多半bare conductors裸导线Permanent faults 永久故障insulator strings 绝缘子串lightning-arrester避雷器ionization电离lightning stroke雷击single line-to-ground faults单相接地故障line-to-line faults两相短路double line-to-ground faults两相接地故障deterioration 退化transient voltage 暂态电压Major insulation 主绝缘adjacent turns 相邻线闸1. 回路系统circuit systemssingle circuit system单回路系统double circuit system双回路系统2. 故障分类types of faultsshort circuit短路open circuit断路permanent fault永久故障temporary fault短时故障3. 对称分量symmetrical components positive-sequence 正序negative-sequence负序zero-sequence零序4. 工频的表示方法power frequency工频working voltage frequency工作电压频率5. 过电压overvoltageexternal overvoltage外部过电压internal overvoltage内部过电压lightning overvoltage雷电过电压switching overvoltage操作过电压transient overvoltage暂态过电压temporary overvoltage临时过电压6. 统计规律statistical lawsrandom distribution随机分布normal distribution正态分布equilibrium 平衡,均衡regain重新达到disturbance 干扰,扰动instability不稳定性manifest表现synchronism 同步性colloquially 用通俗语dynamics动态collapse崩溃issue重点,难题surviving幸免于tie联络线actuate激励,驱使assumption假定,设想formation 形成,构成time span 时间间隔rigorous严格的,严厉的overlap 交迭simulation 仿真analytical 分析的,解析的opposing反向的,相反的torque 扭矩,转矩perturb扰乱,干扰upset 颠覆,推翻deviation背离fluctuation 波动,起伏coefficient系数linearization 线性化permissible 可允许的excursion 偏移,漂移post-disturbance 扰动后的prior to 在前,居先progressive 逐渐的,渐进的profile 轮廓,断面contingency 偶然incremental change 递增,递增量1. 扰动disturbancefault 故障contingency意外事故perturbation 扰动,干扰2. 同步性synchronism synchronous同步的in synchronism同步,保持同步性in step同步out of step 失步asynchronous异步的nonsynchronous非同步的3. 稳定性分类(1) types of stability (1) steady-state stability 稳态稳定性dynamic stability 动态稳定性transient stability暂态稳定性small-disturbance stability小扰动稳定性4. 稳定性分类(2) types of stability (2) synchronous stability同步稳定性angle stability攻角稳定性voltage stability电压稳定性5. 转矩torquessynchronizing torque 同步转矩damping torque 阻尼转矩restoring torque 回复转矩input mechanical torque 输入机械转矩output electrical torque 输出电磁转矩compensation 补偿absorption 吸收excitation 励磁field current 励磁电流end-region 端部armature 电枢surge impedance 波阻抗natural load 自然负荷leakage 漏电lagging 滞后incentive 动机utility 电力单位prolonged 延长的,长时期的adversely 反过来dispersed 分散的sink 接收器synchronous condenser 同步调相机alternative forms 不同形式self-regulating 自调的sunsynchronous 次同步的swing 摇摆cope with 应对,与… 竞争voltage sag 电压暂降thorough 十分的,彻底的deenergized 失磁的,不带电的prescribed 指定的1. 励磁状况excitation status overexcited过励磁的underexcited欠励磁的2. 负荷状况load statusno load空载full load 满载rated load额定负载3. 无功补偿reactive power compensation passive compensation无源补偿active compensation有源补偿4. 无功补偿器reactive power compensatorssynchronous compensator同步补偿器static compensator静止补偿器optimization 最优化profit 利润capital invested 资金投入regulatory body 制定规章的机构conservation 保留,节约kilowatthour 千瓦时unit 发电机组delivered power 输出功率call upon 启用mine-mouth 矿山口preliminary 预备的,初步的auxiliary 辅助设备installation 装置unthinkable 不能想象的rights of way 公共事业用地converter 变流器,换流器mercury-arc 汞弧semiconductor 半导体rectifier整流器inverter 逆变器strip of剥夺line-commutated线换向的respective分别的thyristor晶闸管valve阀bridge arm桥臂trigger触发simultaneously 同时地monopolar 单极的bipolar 双极的homopolar 同极的junction 连接处double-circuit 双回路reversal 颠倒,反向feasible 可行的spare 备用品side effect 副作用cascade 级联的transformer bank 变压器组1. 换流器converterrectifier整流器inverter 逆变器2. HVDC链的分类categories of HVDClinksmonopolar link单极型bipolar link 双极型homopolar link同极型3. HVDC连接HVDC connectionsback-to-back 背对背的point-to-point点对点的multiterminal多端的power electronic-based 基于电力电子static controllers 无静差控制器controllability可控性power transfer capability电力传输能力power electronics 电力电子semiconductor半导体voltage support电压支持power system stabilization电力系统稳定性power quality 电能质量underutilized未利用interconnected network互联系统Static Var Compensator 静止无功补偿装置synchronous compensators调相机thyristor valves 晶闸管阀reactor banks电抗器组in steps 步调一致thyristor switching晶闸管开关fundamental frequency 几波频率branch current 分支电流phase angle 相角firing pulses 点火脉冲firing angle 触发角var output 无功输出nonsinusoidal current非线性电流be tuned to 调谐minimum transients 最小瞬变stepwise 逐步的breakers断路器response time 响应时间is intended as 目的是作为voltage source converters 电压源变换器voltage source inverter 电压源逆变器square-wave 方波in antiparallel to 反并联three-level converter三电平变换器bidirectional 双向potentials 电位semiconductors半导体Static Synchronous Compensator 静止无功补偿器state-of-the-art 最先经的shunt reactive power compensation 并联无功补偿power factor 功率因数power quality电能质量solid-state 使用电子管的equivalent circuit 等效电路accounts for 解释conduction losses传到损耗leakage inductance 漏电感voltage sag电压暂降Active power filters 有源滤波nonlinear loads 非线性负载real time system实时系统fed back to 回馈PWM (Pulse Width Modulation) harmonic components 谐波部分power quality 电能质量deviation 背离,偏移dip 跌落,下垂sag 凹陷,暂降swell 暂升distortion 畸变fluctuation 波动flicker 闪变conditioner 调节装置crest factor 波峰系数Point of Common Coupling (PCC) 公共连接点Root Mean Square (rms) 均方根Total Harmonic Distortion (THD) 总谐波畸变flat-topped 平顶的pointy 尖的saturation 饱和integral multiple 整倍数fundamental 基频的conceivable 可能的,想象到的arbitrary 任意的square wave 方波steep side 陡坡,陡沿pointy corner 尖角decompose 分解magnetic circuit 磁路saturable 可饱和的furnace 炉子dissipate 散失,消耗useful work 有用功net power 净功率resonance 谐振viable alternative 可行的替换物reciprocating 往复的intermittent load 间歇负荷welder 电焊miscellaneous 各种各样的shovel 铲子rolling mill 轧钢厂sensation 感觉illumination 照度unity 单位scrap 废料remedial measure 补救措施advent 出现,到来。
计算机专业英语(张强华-第二版)重点单词及部分课后答案
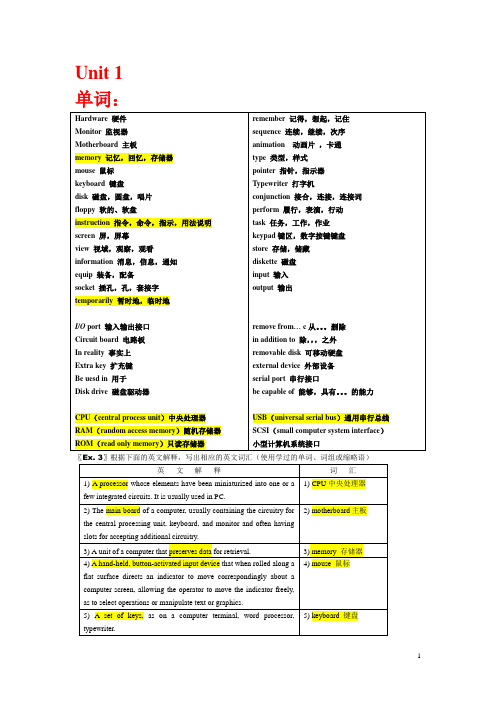
Unit 1单词:〖Ex. 3〗根据下面的英文解释,写出相应的英文词汇(使用学过的单词、词组或缩略语)〖Ex. 5〗把下列短文翻译成中文系统面板和普通键盘有专门控制键,你可以使用这些控制键实现主要的多媒体功能:观相片、听音乐和看电影。
面板还有为看电视和阅读电视指南而设的快速启动按钮。
Ex. 9〗用that从句做宾语将下面汉语译成英语你应该知道,你不仅仅能读取磁盘上的数据,也能够往上面写新的信息1. You should know that you can not only read data from the disk but also write new information to it.你应该意识到,软盘容纳不了多少数据2. You should realize that floppies do not hold too much data.我们计算机老实说,USB要比火线慢多了3. Our computer teacher said that USB is much slower than Firewire.我认为CPU主要责任是执行指令4. I think/believe that the CPU is primarily responsible for executing instructions.Unit 2单词〖Ex. 3〗根据下面的英文解释,写出相应的英文词汇(使用学过的单词、词组或缩略语)Unit 3〖Ex. 3〗根据下面的英文解释,写出相应的英文词汇(使用学过的单词、词组或缩略语)Unit 4单词〖Ex. 3〗根据下面的英文解释,写出相应的英文词汇(使用学过的单词、词组或缩略语)Unit 5单词〖Ex. 3〗根据下面的英文解释,写出相应的英文词汇(使用学过的单词、词组或缩略语)〖Ex. 5〗把下列短文翻译成中文佳能打印机有五种样式,价格从$80 到$500不等,满足了任何想打印相片用户的需求。
Pepperl+Fuchs 14 KFD2-EB2.R4A.B 重复电源模块说明书
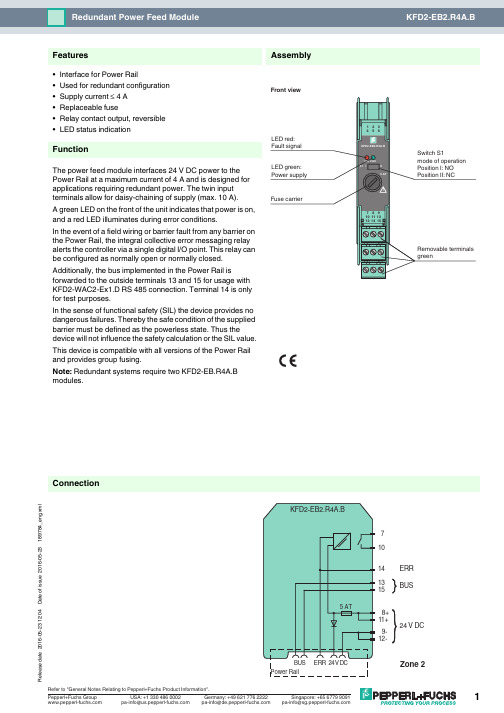
16-05-23 12:04D a t e o f i s s u e 2016-05-23189784_e n g .x m l14131571024 V DC9-12-8+11+BUSERR ConnectionAssembly•Interface for Power Rail•Used for redundant configuration •Supply current ≤ 4 A •Replaceable fuse•Relay contact output, reversible •LED status indicationFunctionThe power feed module interfaces 24V DC power to the Power Rail at a maximum current of 4A and is designed for applications requiring redundant power. The twin input terminals allow for daisy-chaining of supply (max. 10A).A green LED on the front of the unit indicates that power is on, and a red LED illuminates during error conditions.In the event of a field wiring or barrier fault from any barrier on the Power Rail, the integral collective error messaging relay alerts the controller via a single digital I/O point. This relay can be configured as normally open or normally closed.Additionally, the bus implemented in the Power Rail isforwarded to the outside terminals 13 and 15 for usage with KFD2-WAC2-Ex1.D RS 485 connection. Terminal 14 is only for test purposes.In the sense of functional safety (SIL) the device provides no dangerous failures. Thereby the safe condition of the supplied barrier must be defined as the powerless state. Thus the device will not influence the safety calculation or the SIL value.This device is compatible with all versions of the Power Rail and provides group fusing.Note: Redundant systems require two KFD2-EB.R4A.B modules.FeaturesFront view16-05-23 12:04D a t e o f i s s u e 2016-05-23189784_e n g .x mlSupplyConnection terminals 11+, 12-terminals 8+, 9-Rated voltage U n20 ... 30 V DCThe maximum rated operating voltage of the devices plugged onto the Power Rail must not be exceeded.Power dissipation ≤ 2.4 WOutputSupply Output current: ≤ 4 A Fault signal relay output: NO contactContact loading30 V AC/ 2 A / cos φ ≥ 0.7 ; 40 V DC/ 2 A Energized/De-energized delay approx. 20 ms / approx. 20 msFuse rating5 Arecommended maximum utilization of the fuse: 80 %Directive conformity Electromagnetic compatibilityDirective 2014/30/EU EN 61326-1:2013 (industrial locations)ConformityElectromagnetic compatibility NE 21:2006Degree of protection IEC 60529:2001Ambient conditions Ambient temperature -20 ... 60 °C (-4 ... 140 °F)Mechanical specifications Degree of protection IP20Mass approx. 100 gDimensions 20 x 119 x 115 mm (0.8 x 4.7 x 4.5 in) , housing type B2Mountingon 35 mm DIN mounting rail acc. to EN 60715:2001Data for application in connection with Ex-areasStatement of conformityTÜV 00 ATEX 1618 X Group, category, type of protection, temperature class ¬ II 3G Ex nA nC IIC T4Directive conformityDirective 2014/34/EU EN 60079-0:2012+A11:2013 , EN 60079-15:2010International approvals FM approval Control drawing 116-0160Approved for Class I, Division 2, Groups A, B, C, D; Class I, Zone 2, IIC UL approvalApproved for Class I, Division 2, Groups A, B, C, D; Class I, Zone 2, IIC CSA approval Control drawing 116-0160Approved for Class I, Division 2, Groups A, B, C, D; Class I, Zone 2, IIC IECEx approval IECEx UL 16.0051Approved for Ex nA nC IIC T4 Gc General information Supplementary informationStatement of Conformity, Declaration of Conformity, Attestation of Conformity and instructions have to be observed where applicable. For information see .16-05-23 12:04D a t e o f i s s u e 2016-05-23189784_e n g .x mlPower feed module KFD2-EB2The power feed module is used to supply the devices with 24 V DC via the Power Rail. The fuse-protected power feed module can supply up to 150individual devices depending on the power consumption of the devices. Collective error messages received from the Power Rail activate a galvanically-isolated mechanical contact.Power Rail UPR-03The Power Rail UPR-03 is a complete unit consisting of the electrical insert and an aluminium profile rail 35mm x 15mm. To make electrical contact, the devices are simply engaged.Profile Rail K-DUCT with Power RailThe profile rail K-DUCT is an aluminum profile rail with Power Rail insert and two integral cable ducts for system and field cables. Due to this assembly no additional cable guides are necessary.Power Rail and Profile Rail must not be fed via the device terminals of the individual devices!Accessories。
计算机专业英语复习题

一、判断对错1. The software system is the physical equipment that you can see and touch.2. Typically, a data value is set to zero to represent FALSE and 1 value for TRUE.3. In the earliest general-purpose computer, most input and output media were magnetic disks.4. Supercomputers are largest, fastest, and most expensive computer available.5. A computer system consists of hardware system and software system.1. The data bus always receives data from the CPU, and the CPU never reads the data bus.2. Main memory holds whatever programs and data are available for immediate use by theCPU.3. Dynamic RAM does not have to be refreshed.4.Dot-matrix printer work by squirting tiny droplets of liquid ink at the paper.5. The auxiliary memory is very small, relatively expensive, and has very high access speed.1. Shift registers operate in serial fashion all the bits of the word at a time.2. RISC processors have larger instruction sets that often include some particularly complexinstructions.3. SIMD represents an organization that includes many processing units under the supervisionof a common control unit.4. Parallel processing is established by distributing the data among the multiple functional units.5. RISC is a complex instruction set computer.1. A number of different algorithms can exist for solving a computational problem, and each ofthese algorithms could have a same running time complexity.2. The divide-and-conquer algorithm is a bottom-up technique that usually begins by solvingthe smallest subproblems, the dynamic programming solves problems in a top-down fashion.3. An important property of arrays is that their size and shape are constant.4. If all of the elements stored in a list are of the same type, then the list is said to beheterogeneous. However, if different types of elements are stored in the list, then the list is said to be homogeneous.5. A queue is a dynamic set that obeys the LIFO property.1. The computer hardware recognizes only assembly language instruction.2. A program written in the assembly language of one microprocessor can run on a computerthat has a different microprocessor.3. Assembly languages are platform-independent, but high-level languages are notplatform-independent.4. The 4GLs are also called nonprocedural languages.5. Each assembly language instruction corresponds to one unique machine code instruction.二、完形填空Unit 1This chapter introduces digital computer, data types, the evolution of computers, and types of computers. 1 is known to all, it’s hard to find a field in 2 computers are not being used. Digital computer, also called electronic computer or computer, is a digital system that 3 various computational tasks. Digital computers use the 4 number system, which has two digits: 0 and 1.By using various coding 5 , groups of bits can be made to represent not only binary numbers 6 other discrete symbols, such as decimal digits or letters of the alphabet. A computer system consists of hardware system and software system. Programs tell the hardware what to do. 7 software is designed to accomplish real-world tasks in fields such as accounting, entertainment, and engineering. Computers are usually 8 into four broad categories: microcomputers, minicomputers, mainframe computers, and supercomputers. It’s hard to give a 9 definition to each type because computer speeds and storage 10 change rapidly.1. A. As B. It C. As it D. That2. A. what B. which C. where D. when3. A. performs B. carries C. makes D. integrates4. A. decimal B. binary C. Arabian D. American5. A. technique B. technologyC. techniquesD. technologies6. A. instead of B. rather thanC. but alsoD. as well7. A. Application B. System C. Word D. Excel8. A. put B. made C. conducted D. classified9. A. precious B. progress C. proceeding D. precise10. A. capacities B. capable C. capabilities D. capacity1.A2. B3. A4. B5. C6. C7. A8. D9. D 10.AUnit 2A computer system 1 of hardware system and software system. The hardware of the computer is usually divided into three major parts or three 2 subsystems: the CPU, the memory subsystem, and the I/O 3 . The CPU is made up of three major parts, Register Set, the 4 logic unit, or ALU, and Control Unit. It performs many operations and controls computer. Memory is also known as 5 memory or main memory, which is cataloged into two major types of memory: Random Access Memory (RAM) and Read Only Memory (ROM).It refers to the 6 in the computer that hold whatever programs and data are available 7 immediate use by the CPU, along with the program’s data. Computer systems include special hardware 8 between the CPU and peripherals to supervise and synchronize allinput and output transfers. These components are called 9 units because they interface between the processor bus and the peripheral device. The I/O subsystem allows the CPU to 10 with input and output devices.1. A. consists B. makes upC. constitutesD. comprise2. A. premier B. primaryC. preliminaryD. elementary3. A. system B. machine C. subsystem D. device4. A. mathematic B. authorativeC. arithmeticD. authoritative5. A.external B.exterior C. Interior D. internal6. A. circuits B. wires C. lines D. hardware7. A.by B. for C. with D. in8. A.software B. setting C. listing D. components9. A. singular B.dual C. interface D. compact10. A.handle B. interact C. respond D. link1. A2. B3. C4. C5. D6. A7. B8. D9. C 10. BUnit 3In computer engineering, computer architecture is the ___1___ design and fundamental operational structure of a computer system. It is a ___2___ and functional description of requirements, especially speeds and interconnections, and design implementations for the various parts of a computer —___3___ largely on the way by which the central processing unit (CPU) performs internally and accesses addresses in memory. Computer system architecture ___4___ the design of the four ___5___: parallel processing, pipelining, vector processing and RISC. Parallel processing system is used to provide simultaneous data-processing tasks for the purpose of increasing the ___6__ speed of a computer systemand is able to perform ___7___ data processing to achieve faster execution time. A pipeline is a set of data processing elements connected in series, so that the output of one element is the input of the next one. The elements of a pipeline are often ___8___ in parallel or in time-sliced fashion. A vector processor, is a CPU design that is able to run mathematical operations on multiple data elements simultaneously. Computers with vector processing capabilities are in demand in 9___ applications. RISC processors have fewer and __10___ instructions than CISC processors. As a result, their control units are less complex and easier to design.1. A. lastest B. conceptual C. ideal D. simple2. A. plan B. design C. blueprint D. concept3. A. relying B. depending C. attaching D. focusing4. A. refers B. speaks C. involves D. interacts5. A. ingredients B. types C. kinds D. lists6. A. computational B. computerC. computationD. computing7. A. current B. compoundC. concurrentD. massive8. A. displayed B. carriedC. accomplishedD. executed9. A. special B. specialized C. specific D. especial10. A. simple B. simplifying C. singular D. simpler1. B2. C3. D4. C5. A6. A7. C8. D9. B 10.D Unit 4Algorithms are essential to the way computers process information, because a computer program is 1 an algorithm that tells the computer what specific 2 to perform in certain order in order to carry out a speci fied task, such as calculating employees’ paychecks or printing students’ report cards. Thus, an algorithm can be considered to be 3 sequence of operations that can be performed by a turning-complete system. In fact, a data structure is a way of 4 data in a computer so that it can be used efficiently. Often a carefully 5 data structure will allow the 6 efficient algorithm to be used. The choice of the data structure often begins from the choice of an 7 data type.A well-designed data structure allows a variety of critical operations to be performed, using as few resources, both execution time and memory space, as possible. Data structures are implemented by a 8 language as data types and the references and operations they provide. Moreover, different kinds of data structures are 9 to different kinds of applications, and some are highly specialized to certain tasks. For example, B-trees are particularly well-suited for implementation of databases, 10 networks of machines rely on routing tables to function.1. A. essentially B. essential C. partially D. mainly2. A. methods B. steps C. plans D. exercises3. A. some B. a C. the D. any4. A. receiving B. storing C. input D. output5. A. chosen B. madeC. programmedD. picked6. A. very B. most C. more D. less7. A. abstract B.concrete C. special D. certain8. A. basic B. runningC. programmingD. advanced9. A. comforted B. suited C. compared D. hooked10. A. therefore B. with C. when D. while1. A2. B3. D4. B5. A6. B7. A8. C9. B 10. DUnit 5A total computer system includes both hardware system and software system. Hardware consists of the 1 components and all associated equipment. Software refers to the programs that are 2 for the computer. It is possible to be familiar with various3 of computer software without being concerned with details of how the computer hardware operates.A programming language is a language used to write computer programs, which involve a computer 4 some kind of computation or algorithm and possibly control over 5 devices such as printers, robots, and so on. Programming languages differ from 6 languages in that natural languages are only used for interaction between people, while programming languages also allow humans to communicate 7 to machines. Some programming languages are used by one device 8 control another. A prominent 9 of programming languages is to provide instructions to a computer. Thousands of different programming languages have been 10 , and new languages are created every year.1. A. mental B. possible C.essential D. physical2. A. design B. spoken C. writtenD. made3. A. parts B. kinds C.types D. aspects4. A.performed B. to performC. performingD. performs5. A.intenal B. external C. inside D. outside6. A. natural B. Human C. computer D. artificial7. A.orders B. instructions C. codes D. calls8. A. to B. for C. with D. over9. A. show B. research C. purpose D. study10. A. creatingB. created C. to create D. creation1. D2. C3. D4. C5. B6. A7. B8. A9. C 10. B三、汉译英1.coding techniques 编码技术2. application software 应用软件3. floating point data 浮点数据4.timesharing分时,分时技术5. storage capacities 存储容量1. system buses 系统总线2. virtual memory 虚拟存储器3. computer architecture 计算机体系结构4. instruction set 指令集5. direct memory access 直接存储器存取1.parallel processing 并行处理2. pipeline processing流水线处理3. vector processing 向量处理4. scalar processor标量处理器5. backward compatibility 向下兼容1. parallel algorithm并行算法2. exhaustive search穷举搜索3. dynamic programming 动态规划4. doubly-linked list 双向链表5. two-dimensional array 二维数组1. derived class派生类2. Inheritance 继承3. markup languages 标记语言4. Hyperlinks 超链接5. Java virtual machine Java虚拟机四、翻译句子1. By using various coding techniques, groups of bits can be made to represent not only binary numbers but also other discrete symbols.2. System software includes not only the complex programs used by technicians to create application software in the first place but also the organizational programs needed to start up the computer and govern its use of other programs.3. Data are numbers and other binary-code information that are operated on to achieve required computational results.4. Rather than arithmetically or logically manipulating characters, a computer may concatenate strings of characters, replace some characters with others, or otherwise manipulate character strings.5. Software applications like word processing, electronic spreadsheets, database management programs, painting and drawing programs, desktop publishing, and so forth became commercially available, giving more people reasons to use a computer.1. By asserting these internal and external control signals in the proper sequence, the control unit causes the CPU and the rest of the computer to perform the operation needed to correctly process instructions.2. In a computer with virtual memory, less-used parts of programs are shifted from RAM to a hard disk and are moved back only when needed.3. A technique used to compensate for the mismatch in operating speeds is to employ an extremely fast, small cache between the CPU and main memory whose access time is close to processor logic clock cycle time.4. The data transfer rate of peripherals is usually slower than the transfer rate of the CPU, and consequently, a synchronization mechanism may be needed.5. In some computers the interrupt vector is an address that points to a location in memory where the beginning address of the I/O service routine is stored.1. The purpose of parallel processing is to speed up the computer processing capability and increase its throughput, that is, the amount of processing that can be accomplished during a given interval of time.2. It is characteristic of pipelines that several computations can be in process in distinct segments at the same time.3. To achieve the required level of high performance it is necessary to utilize the fastest and most reliable hardware and apply innovative procedures from vector and parallel processing techniques.4. In general, the greater the number of instructions in an instruction set, the larger the propagation delay is within the CPU.5. Although CISC processors are more complex, this complexity does not necessarily increase development costs.1. In short, communication problems arise when the language used for an algorithm's representation is not precisely defined or when information is not given in adequate detail.2. Another common algorithmic structure involves that the need to continue executing a statement or sequence of statements as long as some condition remains true.3. In many algorithms, running time will vary not only for inputs of different sizes, but also for different inputs of the same size.4. Thus, dynamic programming is a bottom-up technique that usually begins by solving the smallest subproblems, saving these results, and then reusing them to solve larger and larger subproblems until the solution to the original problem is obtained.5. The conversion from this conceptual one-dimensional array organization to the actual arrangement within the machine’s memory is straight forward and the data can be stored in a seguence of 24 memory cells with consecutive address in the same order envisioned by the programma.1. Documentation is needed for everyone who will be involved with the program——users, operators, and programmers.2. Rather, programs written in a high-level language or assembly language are converted to machine language, which is then executed by the computer.3. The corresponding programs set forth precise procedures, or series of instructions, and the programmer has to follow a proper order of actions to solve a problem.4. 4GLs may not entirely replace third-generation languages because they are usually focused on specific tasks and hence offer fewer options.5. Inheritance is the means by which objects of a class can access member variables and functions contained in a previously defined class, without having to restate those defi nitions.。
变电一次工程施工作业流程
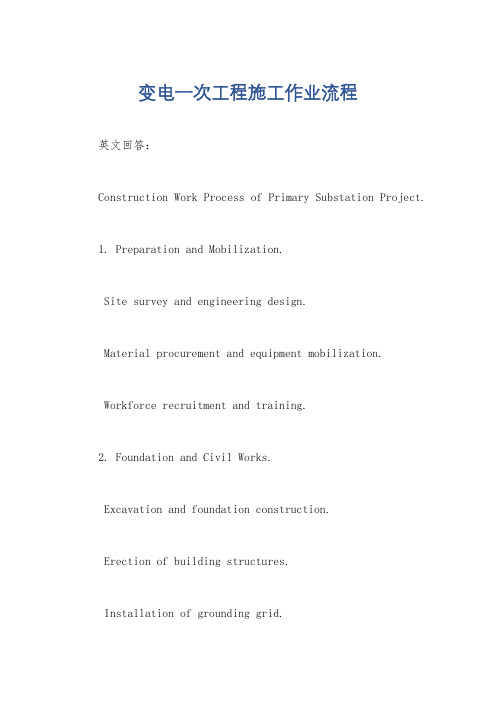
变电一次工程施工作业流程英文回答:Construction Work Process of Primary Substation Project.1. Preparation and Mobilization.Site survey and engineering design.Material procurement and equipment mobilization.Workforce recruitment and training.2. Foundation and Civil Works.Excavation and foundation construction.Erection of building structures.Installation of grounding grid.3. Equipment Installation.Transformer installation and testing.Switchgear installation and testing.Relay and protection system installation.4. Cabling and Interconnections.High-voltage cable installation and termination. Low-voltage cable installation and termination. Busbar installation and connections.5. Commissioning and Testing.Equipment performance testing.System integration testing.Protection and control system testing.6. Operation and Maintenance.Substation energization and handover. Regular maintenance and inspection.Emergency response planning.中文回答:变电一次工程施工作业流程。
F880-RT 8 段冗余字段总线电源供应设备(用于 Yokogawa CENTUM 3000 R3

F880-RT8 Segment Redundant Fieldbus Power Supplyfor use with the YokogawaCENTUM 3000 R3 Control SystemRing Terminal TerminationsF880-RTInstallation InstructionsRelcomCONTENTS PAGE1 OVERVIEW____________________________________________________________12 DESCRIPTION_________________________________________________________13 COMPONENTS AND ACCESSORIES_________________________________________24 MECHANICAL__________________________________________________________34.1 Mounting Orientation________________________________________________3Requirements______________________________________________34.2 EnclosureRequirements____________________________________________34.2.1 GeneralMounting_______________________________________________34.2.2 OutdoorMounting___________________________________________________34.3 DIN-railProcedure______________________________________________34.3.1 Mounting4.4 Removal from DIN-rail_______________________________________________34.5 Mounting and Removal of F801 Power Modules____________________________35 ELECTRICAL CONNECTIONS______________________________________________4Requirements______________________________________________4Power5.1 DC5.2 Power A and Power B________________________________________________45.2.1 Redundancy____________________________________________________55.2.2 Terminator_____________________________________________________55.3 AlarmConnection___________________________________________________5Connections___________________________________________________55.4 HostSegment Connections___________________________________________55.5 Field5.6 Cable Screen / Ground Connections_____________________________________5Ground Connection___________________________________________55.7 Chassis6 TESTING_____________________________________________________________6and Alarm LEDs__________________________________________66.1 F801Status7 ROUTINE MAINTENANCE_________________________________________________68 ATEX CATEGORY 3 INSTRUCTIONS ________________________________________69 CONTROL DRAWING ___________________________________________________7Figure 1: F880-RT1 OVERVIEWThe F880-RT redundant fieldbus power supply is designed to provide redundant power for eight (8) Foundation fieldbus TM H1 segments. Connections are provided on the F880-CA-RT Carrier for two redundant pairs of Yokogawa ALF111 fieldbus cards using Yokogawa AKB336 cables. Power for the eight fieldbus segments is provided by two F801 or F802 Power Modules mounted to the carrier and operating in a redundant configuration. Failure alarms, galvanic isolation, power conditioning and segment termination are incorporated into each Power Module. A single Power Module may be used where redundancy is not required.2 DESCRIPTIONFor maximum reliability, the module carrier contains no components and only provides interconnections between the Power Modules and the external connections. The carrier circuit board is supported in a rigid metal frame that protects it from mechanical damage. Secure DIN-rail mounting is provided by integrated fixings. The Power Module provides galvanic isolation between the 24V DC input power and the fieldbus segments, as required by the IEC61158-2 fieldbus standard and the Fieldbus Foundation TM FF-831 validation test for fieldbus power supplies. There is also galvanic isolation between the fieldbus segments, thereby preventing cross-talk between segments in the event of ground faults in the field wiring. Each segment has its own current limitation and passive fieldbus power conditioner. Termination of the fieldbus segment is automatically maintained when single or redundant Power Modules are fitted. The F880-CA-RT carrier is equipped with connectors that will accept an F809F diagnostic module. The module continuously monitors the performance of each of the eight fieldbus segments, providing information on the network health.Each Power Module has indicator LEDs to show both its status and that of the eight segments under power. In normal operation, each green 'Segment' LED is lit, showingthat the segment is powered. If a segment is shorted, or its voltage is below the rated output, its LED is extinguished, and the red 'Alarm' LED is lit. Redundant 24V DC (nom.) input power is connected to the F880-CA-RT carrier using Ring Terminal connectors.3 COMPONENTS AND ACCESSORIES Product part numbers and their descriptions are given to the right.PART No. DESCRIPTIONF801 8 Segment Power Module: 21.5V, 350mAF802 8 Segment Power Module: 28V, 500mAF809F 8 Segment Fieldbus Diagnostic ModuleF880-CA-RT F880 Ring Terminal CarrierF880-RT F880-CA-RT and two F801 modulesF880-2-RT F880-CA-RT and two F802 modulesF880-RT-NR F880-CA-RT and one F801 moduleF880-2-RT-NR F880-CA-RT and one F802 moduleF800-BLK Blanking Module included with –NR systems4 MECHANICAL4.1 Mounting OrientationThe F880-CA-RT carriers are designed for mounting on a vertically aligned DIN-rail on a vertical surface. This method of mounting ensures optimum heat dissipation from the Power Modules.4.2 EnclosureRequirements4.2.1 General RequirementsThe assembly may be mounted in hazardous (classified) areas – see Sections 8 and 9. The following conditions must also be satisfied to ensure safe and reliable operation.a)Prevent any form of pollution that couldcompromise the operation of the unit. Forexample, choose an unpolluted location or asuitable enclosure to protect the assembly.b)Provide an adequate level of mechanicalprotection. This can be achieved by selecting aprotected location, a suitable enclosure, or acombination of both.c)Ensure that all cable entries and connections aresecure by making provision for the carefulrouting and securing of all cables.d)Provide adequate security against unauthorizedinterference.e)Ensure that the permitted ambient temperaturerange of –40°C to +65°C (derate to +50°C forthe F802 when loaded above 60% of its full loadrating) is not exceeded. Allow for powerdissipation within the enclosure and consider theuse of shading against direct sunlight.4.2.2 Outdoor MountingIf the power system is mounted in an outdoor location, use a suitable enclosure with a minimum of IP54 ingress protection. A higher level of ingress protection rating will be necessary if the working atmosphere is or can be corrosive or if the enclosure is subject to wet or dusty environments.4.3 DIN-railMountingThe carrier is designed for mounting on 35mm x 7.5mm T-section “top hat” DIN rail to EN50022 and uses six built-in DIN clamp tabs to hold it on the rail. The Power Modules must be removed from the carrier to obtain access to the DIN-rail clamp screws. 4.3.1 Mounting ProcedureBefore starting to mount the carrier on the DIN rail, rotate all six of the DIN clamp screws counterclockwise (i.e. unscrew) until the clamping tabs reach the position shown in Figure 3a and none of the tabs extend into the channel for the DIN rail.Press the carrier onto the DIN-rail and rotate each of the DIN clamp screws clockwise until the tab tightens against the DIN-rail. The clamping tabs can be seen through the Inspect clamps holes when they are in the correct position.4.4 RemovalfromDIN-railThe Power Modules must be removed from the carrier to obtain access to the DIN-rail clamp screws. Support the carrier by pressing it to the DIN-rail and unscrew (counterclockwise) the six DIN-rail clamp screws. Turn each screw approximately two full turns when releasing its clamping tab from the rail. Remove carrier.4.5 Mounting and Removal of the PowerModulesThe Power Modules are secured to the carrier with four screw mountings. There are four locating connectors (two for each module) on the module carrier along with mating connectors in the F801 or F802 module. Align the module with the retaining screws and press onto the connector. Hold it in place while tightening the four retaining screws. To remove a Power Module, support the module while unscrewing the four retaining screws at its base. Lift the module off the carrier connector.5 ELECTRICALCONNECTIONSThe DC power, Alarm, and Field Segment connections are all made using Ring terminals on the barrier strip. The barrier strip will accommodate up to 8mm diameter Ring terminals with hole sizes from 3.5mm to 5.0mm.The Field Segment connections do not include a terminal for the cable shield. Figure 5 illustrates how the segment cable should be terminated on the barrier strip. The shield (screen or drain) wire should be cut off in a manner that will not allow it to short out to any metal or terminals. Alternatively, if the shield wire must be grounded in the same cabinet as the F880-CA-RT, the user must supply a method to accomplish this.Note: All fieldbus segments must have the shield grounded at only one place. Because different users want to accomplish this in different ways, we did not provide a terminal for landing the shield wires.Figure 5: Ring Terminal Attachment5.1 DCPowerRequirementsDual redundant power terminals requiring a nominal input voltage of 24VDC are provided allowing the use of bulkpower supplies with a supply range of 19.2–30VDC. Input power cabling and over-current protection devices must be chosen to match the current consumption.An F880 system, operating with redundant F801 modules at24VDC, will require 3.5A in total if all eight segments drawthe maximum of 350mA. With redundant F802 modules the system will draw 6A when all segments are loaded to 500mA. Note that the total current could be drawn from either the Power A or Power B connection if one power module is removed.It is also important to size the Bulk Power Supply to handlethe inrush current required by the F801 or F802. Inrush isless than 2 times the maximum rated current for the F801with a duration lasting less than 1ms. For the F802, theinrush is less than 4 times the maximum rated current with a duration less than 10ms.5.2 Power A and Power BPower A supplies Power Module A and Power B suppliesPower Module B. Each module provides DC power to all eight segments - see Figure 4. DC supply connections are as follows:Power A Power BPin No. Connection Pin No. Connection1 +ve 41 +ve2 -ve 42 -veCable lengths on the Power connectors should not exceed30m.5.2.1 RedundancyFor redundant operation, Power Modules must be fitted in Power Module A and Power Module B locations. For non-redundant operation fit an F801 or F802 in the Power Module A location and apply power to Power A terminals. The F800-BLK would then be placed on the Power Module B connector to allow the alarm circuit to function. Power must be connected to Power B terminals if live replacement of Power Module A is necessary since Power B terminals feed the Power Module B connector.5.2.2 TerminatorA single terminator is provided automatically on each segment whether one or two Power Modules are used.5.3 AlarmConnectionIn an alarm condition a normally closed, galvanically-isolated relay contact in each Power Module goes to an open-circuit condition. These relays are connected in series on the F880-CA-RT carrier and are presented at terminals as follows:AlarmPin No. Connection91 +ve92 -veIn non-redundant applications using a single Power Module, the alarm connections on the carrier will be open-circuit, indicating a permanent alarm condition unless the F800-BLK module is fitted in place of the missing Power Module.If multiple F880 units are used, a common alarm circuit can be achieved by 'daisy-chaining' the alarm circuits (see Figure 6). 5.4 HostConnectionsConnectors are provided to permit direct connection viaAKB336 cables to Yokogawa ALF111 cards. Four connectorsare fitted to provide the following connections. The hostcable length should not exceed 30 meters.Connector Function Segments Host 1A Main 1 – 4Host 1B Redundant 1 – 4Host 2A Main 5 – 8Host 2B Redundant 5 – 85.5 FieldSegmentConnectionsThere are eight field segment connectors. Each connector provides (+) and (–) connections. No cable screenconnections are provided on the F880-CA-RT carrier.Pin No. Connection Pin No. Connection82 Segment 1 + 70 Segment 5 +84 Segment 1 - 72 Segment 5 -79 Segment 2 + 67 Segment 6 +81 Segment 2 - 69 Segment 6 -76 Segment 3 + 64 Segment 7 +78 Segment 3 - 66 Segment 7 -73 Segment 4 + 61 Segment 8 +75 Segment 4 - 63 Segment 8 -5.6 Cable Screen / Ground ConnectionsIf the screens of the fieldbus cables are required to beearthed in the equipment cabinet housing the F880 system, a separate earth bar, connected to local instrumentation earth, should be used. Note that the Yokogawa AKB336 cables donot carry segment screens. Screen grounding as recommended by the standards must be done downstream ofthe F880 field connectors – either in the F880 equipmentcabinet or a marshalling cabinet.5.7 GroundConnectionsThere are two Ground connections on the carrier. Terminal51 is internally connected to the chassis of the carrier and should be wired to a Safety or Instrumentation Ground. This ground is needed for the F880 system to meetElectromagnetic Compatibility and Electrical Safety requirements. Terminal 52 must be grounded to the same potential as the Fieldbus screens if the F809F diagnosticmodule is used. This Signal Ground connection is used bythe F809F to detect screen shorts to the Fieldbus wiring.6 TESTING6.1 F801 / F802 Status and Alarm LEDs Each Power Module is fitted with nine LEDs, eight to indicate segment status and one to signify an Alarm condition.Fault Condition SegmentLED FaultLEDAlarm contactstatusNo Fault Green Off Closed Segment shorted Off Red Open Output <20V Off Red Open Input <16V Off (All) Red Open 7 ROUTINEMAINTENANCECheck the general condition of the installation occasionally to make sure that no deterioration has occurred. At least every two years (and more frequently for particularly harsh environments) check:∙the condition of wire connections, terminations, and screens.∙the dc output voltage on each of the eight fieldbus segments is >21.5V for the F801 or >28V for theF802. This can be performed using a multi-meter ora Relcom FBT-3/FBT-6 Fieldbus Monitor.∙the segment LEDs on the Power Module(s) are functioning and the Alarm LED is not lit .∙the Power Module retaining screws are tight.∙there are no signs of damage or corrosion.8 ATEX CATEGORY 3 INSTRUCTIONSSafety instructions for installation and operating personnelThis manual contains basic safety instructions for installation, operation and maintenance and servicing. Failure to comply with these instructions can endanger personnel, the plant and the environment.Before installation/commissioning:∙Read the operating instructions.∙Give adequate training to the installation and operating personnel.∙Ensure that the contents of the operating instructions are fully understood by responsible personnel.∙The national installation and mounting regulations (e.g. EN 60079-14, National Electrical Code) apply.When operating the apparatus:∙Make the operating instructions available at the installation area (at all times).∙Observe safety instructions.∙Observe national safety and accident prevention regulations.∙Operate the equipment within its published specification.Servicing/maintenance work or repairs which are not described in the operating instructions must not be performed without prior agreement with the manufacturer.Any damage may render explosion protection null and void.No changes to the devices or components impairing their explosion protection are permitted.The device may only be fitted and used if it is in an undamaged, dry and clean state.9 CONTROLDRAWING2221 Yew Street, Forest Grove, Oregon 97116 USA。
Fulcrum IMSAI兼容S-100微计算机系列产品说明书
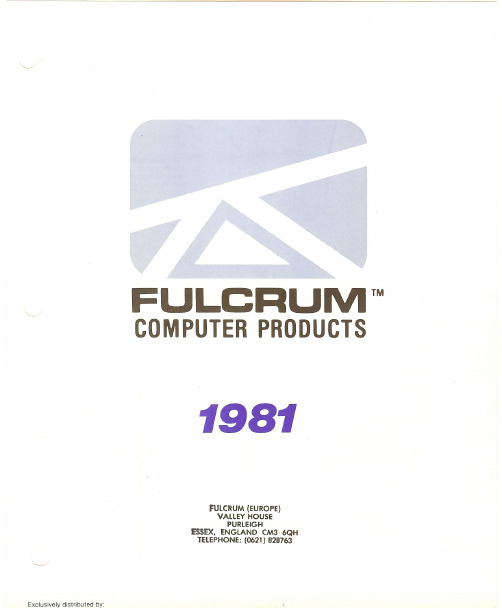
and +8V supply voltages and uses on-board regulators to obtain required voltage levels.
The board edge connector has 100 pins on 0.125-inch centers, with 50 pins on each side. Except for goldplated contact fingers, circuit traces are tin-lead plated for easier, more reliable solder connections.
Front Panel
• Handsome and functional, with sharp, readable legends behind acrylic panel
• All indicators long-life LED's ... panel filter enhances contrast
Motherboard (WB20) • Documentation
Motherboard
Card-to-card spacing on the Mother Board is %-inch, except for the first position reserved for the front panel board or any other board in dedicated applications. Eight, ten, twelve and twenty slot terminated Mother Boards are available for the system, good to 10 MHz.
IEEE P2030.11 WG Distributed Energy Resources Mana
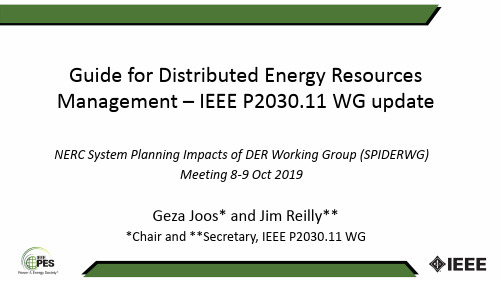
Guide for Distributed Energy Resources Management –IEEE P2030.11 WG update Geza Joos* and Jim Reilly***Chair and **Secretary, IEEE P2030.11 WGNERC System Planning Impacts of DER Working Group (SPIDERWG)Meeting 8-9 Oct 2019Topics addressed•Distributed Energy Resources (DER) –Interconnection at D and T levels –microgrids, VPP and DERMS•DER characteristics and features•Flexibility requirements and DER as service providers•DER aggregation –DERMS services –IEEE Std 2030.11•DERMS architecture and functions/services•IEEE Std 2030 smart grid series –interoperability requirementsDER in active D grids and T gridsTransmission gridCentral power plantsSolar farms Microgrid – gridconnected Substation Virtual PowerPlant (VPP)Distribution gridWind farms Demandresponse Loads Electrical storage Distributed generationresources (DER)SubstationDistributed generation Demand response Electrical storage Micro/gas turbines/CHP DER Renewables Wind/solar Loads Substation Aggregated DERDER features –types and features •Energy resource –local power generation–Local resources –solar, wind –renewables non dispatchable–Conventional fuels (diesel engines)•Storage –generator (discharging) / load (charging)–Firming production –balancing variability–Leveraging –storing/retrieving excess power•Controlled/curtailable loads –demand response •Large scale deployment requires DER support to T&D gridsT grid flexibility requirements and resourcesNet load fluctuations Power system elementsNeed for flexibility Resources for flexibilityDemandvariability Renewable DER variabilityContingencies Power marketsSystemoperationGrid hardwarePower plants /DERDemandresponse / DEREnergy storage /DERInterconnectionsResources providing flexibility –DER typesResources providing operational flexibilityPower generation - conventional RenewableswInd-solar /DERFully dispatchable Residential loads Demand Response /DER Energy storage / DER Batteries, hydro, flywheel, CAES Electric vehicles, other Time dependentavailability Thermal loads, HVAC Industrial loadsFully controllable [+/-] (load, generator)Fully controllable [+/-] (when connected)Controllable -interruptible IndustrialprocessesControllable -interruptibleFully controllable [+/-] (Power, energy)InterruptibleLimited control [+/-]DER impact on T grids•DER may represent a significant portion of power and energy produced as renewable resources penetration increases •Impacts on T operation–Generation dispatch –non dispatchable and balancing requirements –Reduction in system inertia –inverter based resources–Protection –reduction in short circuit capacity•Required –approaches for management of 100,000 + devices •Interface between DER and grids –aggregators and DERMSIEEE P2030.11 –DERMS guide •Distributed Energy Resources Management Systems (DERMS) Functional Specification–Purpose of DERMS –aggregation of DER to provide system services •DERMS –interface between aggregated DER and DMS, TSO •Scope of Guide–Guiding principles for application and deployment of DERMS–Functional requirements and core functions–Defining functions to enable system servicesRole of the DERMS as aggregatorTransmission grid Demandresponse Loads Electrical storage Generation Distributed generation DERPortion of the distribution gridDistribution gridSubstationTSO / EMS DSO / DMSDERMSIEEE P2030.11 –DERMS core functionsG.J.Higher level functions Operator interface Communications/SCADAGrid/market Optimal dispatch Core level functions Aggregation – DER device information – registration, groupingDER/DERMS monitoring and status – dynamic grouping DERMS operation and control – dispatch, estimationL e v el 3L e ve l2Lower level functionsVoltage/frequency control Device specific functions Real/reactive power controlL e v e l 1DER devices and associated components Electric grid – distribution / transmissionDERMS control system DMS TSO ISO DER control systemDERMS core functions and groupingsDER/DERMS monitoring/status Status/alarms Dynamic groupingMeasurements/ weather DER device informationGrouping CapabilityRegistration DERMS operation/controlControl/optimization*Estimation/forecasting*Dispatch/scheduling Vizualization*Historian*System services enabled by DERMS •Basic energy and capacity –meeting needs•Frequency response, support and control –also includes –Ramping services–Inertial response, post contingency frequency support•Voltage regulation, support and control –also includes –Maintaining/setting voltage profile for optimizing T system operation •Black start in support of power system restorationIEEE Std 2030.n smart grid series •IEEE Std 2030 –general smart grid interoperability guide –in force•IEEE Std 2030.2/3 –battery energy storage –in force •IEEE Std 2030.7/8 –microgrid controllers –in force •IEEE P2030.4 –interoperability –WG•IEEE P2030.11 –DERMS specification –WGIEEE Std 2030 –Smart Grid Interoperability •Proposing a SG Interoperability Reference Model (SGIRM)•Providing a knowledge base for terminology, characteristics, and smart grid functional performance •Defining 3 integrated architectural perspectives –power, communications, and information technologies •Defining functional interfaces –logical connections –data flow characteristics (ICT, security /cyber)。
TJA1054中文资料
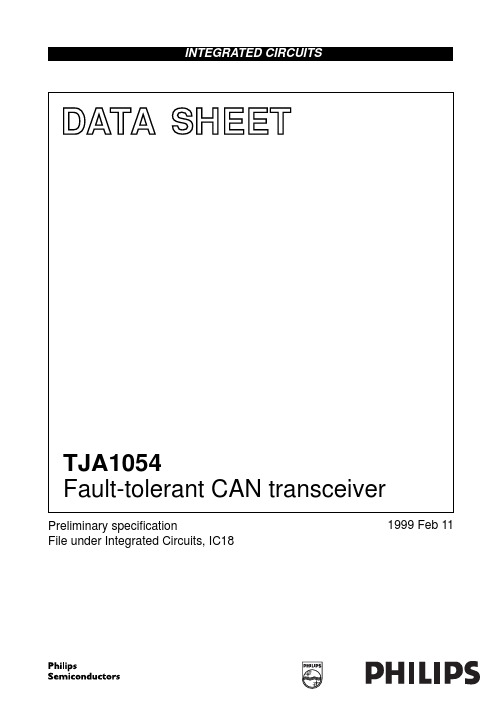
Philips Semiconductors
Preliminary specification
Fault-tolerant CAN transceiver
PINNING SYMBOL INH TXD RXD ERR STB EN WAKE RTH RTL VCC CANH CANL GND BAT PIN 1 2 3 4 5 6 7 8 9 10 11 12 13 14 DESCRIPTION
元器件交易网
INTEGRATED CIRCUITS
DATA SHEET
TJA1054 Fault-tolerant CAN transceiver
Preliminary specification File under Integrated Circuits, IC18 1999 Feb 11
VERSION SOT108-1
1999 Feb 11
2
元器件交易网
Philips Semiconductors
Preliminary specification
Fault-tolerant CAN transceiver
QUICK REFERENCE DATA SYMBOL VCC VBAT PARAMETER supply voltage on pin VCC battery voltage on pin BAT no time limit operating mode load dump IBAT VCANH battery current on pin BAT CANH bus line voltage CONDITIONS MIN. 4.75 −0.3 5.0 − − − − − 30 − TYP.
datasheetpreliminaryspecificationfileunderintegratedcircuitsic181999feb11integratedcircuitstja1054faulttolerantcantransceiver元器件交易网wwwcecb2bcom1999feb112philipssemiconductorspreliminaryspecificationfaulttolerantcantransceivertja1054featuresoptimizedforincarlowspeedcommunication?baudrateupto125kbaud?upto32nodescanbeconnected?supportsunshieldedbuswires?verylowradiofrequencyinterferencerfiduetobuiltinslopecontrolfunctionandaverygoodmatchingofthecanlandcanhbusoutputs?fullyintegratedreceiverfilters?permanentdominantmonitoringoftransmitdatainput?goodimmunityperformanceofelectromagneticcompatibilityemcinnormaloperatingmodeandinlowpowermodesbusfailuremanagement?supportssinglewiretransmissionmodeswithgroundoffsetvoltagesupto15v?automaticswitchingtosinglewiremodeintheeventofbusfailuresevenwhenthecanhbuswireisshortcircuitedtovcc?automaticresettodifferentialmodeifbusfailureisremoved?fullywakeupcapabilityduringfailuremodesprotection?shortcircuitprooftobatteryandgroundin12vpoweredsystems?thermallyprotected?buslinesprotecte
CIGRE BENCHMARK MODEL
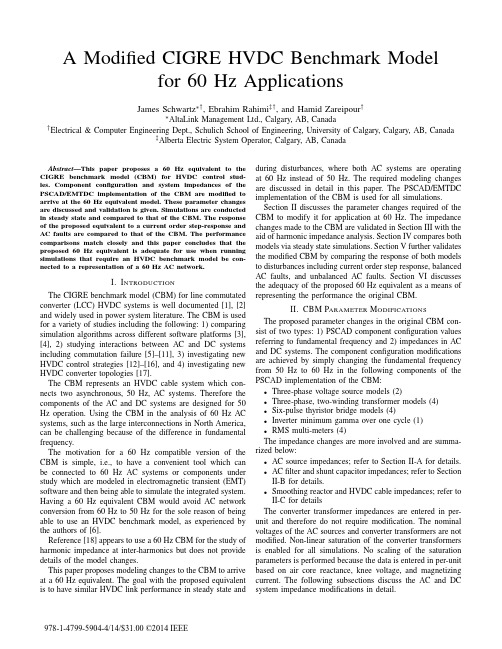
A Modified CIGRE HVDC Benchmark Modelfor60Hz ApplicationsJames Schwartz∗†,Ebrahim Rahimi‡†,and Hamid Zareipour†∗AltaLink Management Ltd.,Calgary,AB,Canada†Electrical&Computer Engineering Dept.,Schulich School of Engineering,University of Calgary,Calgary,AB,Canada‡Alberta Electric System Operator,Calgary,AB,CanadaAbstract—This paper proposes a60Hz equivalent to the CIGRE benchmark model(CBM)for HVDC control ponent configuration and system impedances of the PSCAD/EMTDC implementation of the CBM are modified to arrive at the60Hz equivalent model.These parameter changes are discussed and validation is given.Simulations are conducted in steady state and compared to that of the CBM.The response of the proposed equivalent to a current order step-response and AC faults are compared to that of the CBM.The performance comparisons match closely and this paper concludes that the proposed60Hz equivalent is adequate for use when running simulations that require an HVDC benchmark model be con-nected to a representation of a60Hz AC network.I.I NTRODUCTIONThe CIGRE benchmark model(CBM)for line commutated converter(LCC)HVDC systems is well documented[1],[2] and widely used in power system literature.The CBM is used for a variety of studies including the following:1)comparing simulation algorithms across different software platforms[3], [4],2)studying interactions between AC and DC systems including commutation failure[5]–[11],3)investigating new HVDC control strategies[12]–[16],and4)investigating new HVDC converter topologies[17].The CBM represents an HVDC cable system which con-nects two asynchronous,50Hz,AC systems.Therefore the components of the AC and DC systems are designed for50 Hz ing the CBM in the analysis of60Hz AC systems,such as the large interconnections in North America, can be challenging because of the difference in fundamental frequency.The motivation for a60Hz compatible version of the CBM is simple,i.e.,to have a convenient tool which can be connected to60Hz AC systems or components under study which are modeled in electromagnetic transient(EMT) software and then being able to simulate the integrated system. Having a60Hz equivalent CBM would avoid AC network conversion from60Hz to50Hz for the sole reason of being able to use an HVDC benchmark model,as experienced by the authors of[6].Reference[18]appears to use a60Hz CBM for the study of harmonic impedance at inter-harmonics but does not provide details of the model changes.This paper proposes modeling changes to the CBM to arrive at a60Hz equivalent.The goal with the proposed equivalent is to have similar HVDC link performance in steady state and during disturbances,where both AC systems are operating at60Hz instead of50Hz.The required modeling changes are discussed in detail in this paper.The PSCAD/EMTDC implementation of the CBM is used for all simulations. Section II discusses the parameter changes required of the CBM to modify it for application at60Hz.The impedance changes made to the CBM are validated in Section III with the aid of harmonic impedance analysis.Section IV compares both models via steady state simulations.Section V further validates the modified CBM by comparing the response of both models to disturbances including current order step response,balanced AC faults,and unbalanced AC faults.Section VI discusses the adequacy of the proposed60Hz equivalent as a means of representing the performance the original CBM.II.CBM P ARAMETER M ODIFICATIONSThe proposed parameter changes in the original CBM con-sist of two types:1)PSCAD component configuration values referring to fundamental frequency and2)impedances in AC and DC systems.The component configuration modifications are achieved by simply changing the fundamental frequency from50Hz to60Hz in the following components of the PSCAD implementation of the CBM:•Three-phase voltage source models(2)•Three-phase,two-winding transformer models(4)•Six-pulse thyristor bridge models(4)•Inverter minimum gamma over one cycle(1)•RMS multi-meters(4)The impedance changes are more involved and are summa-rized below:•AC source impedances;refer to Section II-A for details.•ACfilter and shunt capacitor impedances;refer to Section II-B for details.•Smoothing reactor and HVDC cable impedances;refer to II-C for detailsThe converter transformer impedances are entered in per-unit and therefore do not require modification.The nominal voltages of the AC sources and converter transformers are not modified.Non-linear saturation of the converter transformers is enabled for all simulations.No scaling of the saturation parameters is performed because the data is entered in per-unit based on air core reactance,knee voltage,and magnetizing current.The following subsections discuss the AC and DC system impedance modifications in detail.978-1-4799-5904-4/14/$31.00 ©2014 IEEEA.AC Source ImpedancesThe values of short-circuit ratio(SCR)and damping angles specified in[1]should be maintained in the modified CBM so that HVDC performance is similar to the original CBM.The definition of SCR[19]is given by(1):SCR=SCMV AP d=V2ratedP d|Z th|=V2ratedP dR2th+(ω1L th)2(1)where SCMV A is the short-circuit MV A of the AC system. P d is the rated HVDC link capacity.Z th is the Thevenin impedance seen from the converter AC bus,evaluated at fundamental frequency.Expressing Z th in rectangular form, as shown in(1),permits manipulation of the key parameter for this paper,i.e.,the system fundamental frequencyω1. Let a constant k be defined to represent the change in fundamental frequency required for the modified CBM:ωnew=f new2π=f old2πf newf old=ωoldf newf old=kωold(2)where allωand f values are fundamental frequencies of their respective system.For the development of the modified CBM,k=ω1new /ω1old=60/50=1.20.Therefore in order tokeep SCR constant,L th in(1)must be replaced with L th/kto counteract the replacement ofω1old withω1new=kω1old.Therefore,all inductances in the source impedance are divided by k=1.2,and resistances are not changed.It follows that the damping angles will also be preserved.B.AC Filters and Shunt CapacitorsThe procedure used in section II-A is also used to modify the ACfilter and shunt capacitor impedances as follows:•Inductances divided by k=1.2.•Capacitances divided by k=1.2.•Resistances not changed.Since a general capacitor impedance is given byZ=1/(jωC),all capacitances in the modified CBM must be divided by k=1.2for the same reason as with the inductances in Section II-A.C.Smoothing Reactor and HVDC CableA unique feature of the CBM is the DC-side resonance at fundamental frequency and AC-side resonance at second harmonic[1],[2].Reference[20]discusses how the HVDC cable can be replaced by an equivalent overhead transmission line model,in which this DC-side resonance at fundamental frequency is preserved.The parameters of the smoothing reac-tor,cable resistance,and cable capacitance must be considered here because the fundamental frequency is being changed ac-cording to(2).In similar fashion to the ACfilters as discussed in Section II-B,the values of the smoothing inductance and cable capacitance are each divided by k=1.2in the modified CBM.The value of the cable resistance is not changed.|Z+|[Ω]Fig.1.Harmonic impedance scan at rectifier ACbus|Z+|[Ω]Fig.2.Harmonic impedance scan at inverter AC busIII.V ALIDATION OF I MPEDANCE C HANGESTo validate the AC-side impedance changes proposed in Section II,a harmonic impedance scan is performed at each converter AC bus in both the original CBM and the modified CBM.For harmonic ranks from0to50,the magnitude of positive sequence impedance is recorded.The harmonic impedance comparison for rectifier and inverter is shown in Fig.1and Fig.2,respectively.The AC harmonic impedance comparison shows agreement between the original and modified CBM,which is expected. The harmonic impedance scans show a pronounced harmonic resonance at second harmonic which agrees with[1],[2].A Thevenin impedance calculation,not shown in this paper,of the smoothing reactor in parallel with the cable capacitance shows a peak impedance at fundamental frequency in both the original and modified CBM.IV.S TEADY S TATE C OMPARISONThe original and modified CBMs are simulated in PSCAD/EMTDC and brought to steady state with a current order of1.0pu.Various AC and DC variables were measured and recorded for comparison.The results are shown in Table I,recorded at a simulation time of t=2.0s.TABLE IS TEADY S TATE S IMULATION R ESULTSOriginal CBM Modified CBM error[%] V ac rec[pu,rms] 1.013 1.0140.10P ac rec[MW]1013.721015.280.15Qac rec[Mvar]-46.38-47.78 3.02V ac inv[kV rms]0.9910.9920.10P ac inv[MW]959.78960.70.10Qac inv[Mvar]76.778.41 2.23αrec[◦]20.1220.150.15γinv[◦]15.1715.170V d rec[kV]497.83498.280.09Id[kA]220V d inv[kV]488.74489.290.11 The reader should note that some of the variables in Table I are not truly constant during steady state.For example,the firing angle,αrec,is observed in both the original CBM and modified CBM to vary with a range of0.3◦during what is con-sidered steady state.This will affect the bus voltage and power measurement when compared at a particular time step.Id and γinv have zero percent error because the rectifier and inverter are in constant current(CC)and constant extinction angle (CEA)control modes,respectively.The proportional-integral (PI)controllers ensure that the controlled value matches the setpoint value with zero error during steady state.The steady state results comparison in Table I show agree-ment between both models with all errors being less than3.0%, and the majority of errors being less than0.2%.Therefore comparison of EMT performance may proceed with reason-able confidence in the base electrical model.V.E LECTROMAGNETIC T RANSIENT C OMPARISON Most HVDC projects are delivered to manufacturers who must procure the equipment according to a performance-based technical specification[21].In light of this,the EMT comparisons given in the following subsections are compared from the perspective of recovery duration after disturbances are cleared.The disturbances analyzed are a current order step response, three-phase AC faults,and1-phase AC faults.All of the AC faults are applied on the source-side of the converter transformers.For all disturbances,the original CBM(50Hz) and the modified CBM(60Hz)are simulated separately and the relevant channels are recorded.MATLAB is then used to combine and plot related channels on a common horizontal axis of cycles elapsed after the disturbance is applied,i.e.,each plot in the following subsections shows the same variable as simulated by the original CBM and the modified CBM. Output channels from the original CBM and modified CBM are best compared on a common axis of number of cycles instead of a common time axis because the two systems have different fundamental frequencies.If channels are compared on a common time axis,the channels from the60Hz CBM will appear as an advanced version,in time,of those from the 50Hz CBM.The time-shift between these two channels is not constant,but increases with time according to the difference in fundamental frequencies,i.e.,in the60Hz system each cyclenumber of cycles after current order increase requestFig.3.Rectifier channels during current order step-responseof the AC system voltage occurs(1/50)−(1/60)=3.33ms faster than in the50Hz system.The selected output channels for plotting in the next sub-sections are the following:•rectifier measured direct-current(CMR),Id rec•rectifierfiring angle(ALFA REC),αrec•inverter measured direct-current(CMI),Id inv •inverter extinction angle(GAMA INV),γinvThe reason for this is because in the CBM the rectifier is in CC control mode while the inverter can be in either CEA or CC control mode.Therefore,by analyzing the output channels for Id,α,andγwe should be observing the most critical channels for EMT performance comparisons.A.Direct Current Step-ResponseThe PSCAD/EMTDC implementation of the CBM does not contain a lag/smoothing block between the current order from the user and the PI controller used for calculation of the converterfiring angles.Therefore,a sudden change in the current order can result in a sudden change infiring angle. This can result in a sudden increase of direct current which, if large enough,will cause commutation failure(CF)of the inverter[5].The smoothing reactors help limit the rate of rise of Id,however not enough by which to completely eliminate CF.For this comparison,both simulations are brought to steady state with Id=0.8pu.A current order increase of0.2pu was requested at thefirst zero-crossing of the rectifier phase A voltage,after t=1.4s.Thus,the channels from the both models start at the same point-on-wave.Output channels from the rectifier and inverter are shown in Fig.3and Fig.4, respectively.The step change is requested at cycle zero.αrec andγinv are observed to decrease slightly to allow the initial increase of Id. The inverter CEA controller acts to reduceαinv in an effort to mitigate the decrease ofγinv.This is not successful because the increasing Id results in an increasing inverter overlapnumber of cycles after current order increase requestFig.4.Inverter channels during current order step-responsenumber of cycles after fault applicationFig.5.Rectifier channels during a3-phase,4-cycle,AC rectifier fault angle,µinv,which results inγinv continuously reducing until CF occurs as shown in Fig.4b at approximately1cycle. The reader should recall that the sum of angles for a given converter must be180◦at any time[5],i.e.,α+µ+γ=180◦. The important point to note from the comparisons in Fig. 3and Fig.4is that the output channels are similar and they reach the same values for the new steady state.B.Rectifier Balanced AC FaultA3-phase,4-cycle,rectifier AC fault is applied to both models with Id=1.0pu.The rectifier and inverter channels for both models are shown in Fig.5and Fig.6,respectively. After fault clearing,HVDC recovery begins and CF occurs at approximately12.5cycles after fault application:γinv is observed to drop to zero when the inverter control switches from CC to CEA mode.This CF resolves itself and the HVDC begins recovery to the pre-fault current order of1.0pu.The comparison of the channels from both models match until approximately12.5cycles after fault application.CF occurs faster in the original CBM than the modified CBM,asnumber of cycles after fault applicationFig.6.Inverter channels during a3-phase,4-cycle,AC rectifier faultnumber of cycles after fault applicationFig.7.Rectifier channels during a3-phase,4-cycle,AC inverter fault shown byγinv reaching zero in Fig.6b.After this occurs,the response of both models is the same but the channels from the original CBM are advanced from those of the modified CBM.The reason why CF occurs faster in the original CBM is attributed to the controller parameters not being changed in the modified CBM,i.e.,time-constants and gains used in the inverter CEA and CC controllers.Despite this transient shift in the output channels for a few cycles,both models reach the same Id values with the same duration,i.e.,approximately20 cycles after fault application,as shown by Fig.5a and Fig.6a.C.Inverter Balanced AC FaultA3-phase,4-cycle,inverter AC fault is applied to both models with Id=1.0pu.The rectifier and inverter channels for both models are shown in Fig.7and Fig.8,respectively. The inverter balanced AC fault causes the AC and DC voltage to drop to zero and the inverter experiences CF. After the AC fault is cleared,both models start recovery andFig.8.Inverter channels during a3-phase,4-cycle,AC inverter faultnumber of cycles after fault applicationFig.9.Rectifier channels during a1-phase,4-cycle,AC rectifier fault experience a second CF.This is observed byγinv reaching zero in both models for a second occurrence;refer to Fig.8b. The comparison of the channels from both models match until approximately7cycles after fault application.The second CF occurs faster in the original CBM than the modified CBM. As in Section V-B,this is attributed to the inverter controller parameters not being changed in the modified CBM.All output channels shown in Fig.7and Fig.8match again at approximately20cycles after fault application.The difference in the output channels between7cycles and20 cycles after fault application is more pronounced than that observed in Section V-B.Theαrec andγinv channels appear to have a larger difference than the Id channels.D.Rectifier Unbalanced AC FaultA phase-A,4-cycle,rectifier AC fault is applied to both models with Id=1.0pu.The rectifier and inverter channels for both models are shown in Fig.9and Fig.10,respectively. Because of the bolted fault placed on phase A at the rectifier AC bus,the rectifier loses control of the current as shownbynumber of cycles after fault applicationFig.10.Inverter channels during a1-phase,4-cycle,AC rectifier fault αrec reaching its minimum value of5degrees.The inverter takes over control of the current,as supervised by the voltage-dependent current-order limit(VDCOL).This can be observed by the significant increase inγinv,and Id inv being controlled to approximately0.6pu.After the fault is cleared,voltage at the rectifier AC bus returns to normal and the limitation onαrec is released,i.e.,the rectifier resumes control of the current at the pre-fault current order of1.0pu.The comparison of the channels from both models match until approximately6cycles after fault application.γinv in the original CBM drops very low at the6cycle mark whereas that in the modified CBM does not follow this excursion.Despite this transient difference,all channel values in Fig.9and Fig. 10match once again at15cycles after fault application.This transient difference is attributed to no controller modifications being made in the modified CBM.E.Inverter Unbalanced AC FaultA phase-A,4-cycle,inverter AC fault is applied to both models with Id=1.0pu.The rectifier and inverter channels for both models are shown in Fig.11and Fig.12,respectively. As with the balanced inverter fault discussed in Section V-C, the drop in inverter AC bus voltage at fault application results in CF.After the fault is cleared,the inverter AC voltage returns to nominal and the HVDC link recovers from the fault without any subsequent CFs.This is unlike the case of a balanced AC fault at the inverter which results in a second CF during the recovery,as discussed in V-C.The channels from both models are similar throughout the simulation.No transient difference is observed in Fig.11and Fig.12as with the previous EMT simulations in this paper.VI.D ISCUSSIONThe steady state comparisons in Section IV indicate the modified CBM resembles that of the original within3.0% error.The EMT simulations in Section V show that the mod-ified CBM response to typical disturbances closely matches that of the original when compared on a per-cycle basis.Thenumber of cycles after fault applicationFig.11.Rectifier channels during a1-phase,4-cycle,AC inverterfaultFig.12.Inverter channels during a1-phase,4-cycle,AC inverter fault comparisons match during the fault.Some transient differences are observed after fault clearing.The same steady state is reached by both models with the same duration,i.e.,number of cycles.The performance of the modified CBM could be made closer to that of the original if the converter control time-constants and gains are studied and modified.Given the simple modifications indicated in Section II,the performance of the modified CBM as reported in this paper meets the goal stated in Section I,i.e.,a convenient tool with similar performance to the original CBM.VII.C ONCLUSIONThis paper presents simple component and impedance changes to the PSCAD/EMTDC implementation of the CIGRE Benchmark Model(CBM)which effectively convert it to a60 Hz equivalent.The impedance modifications are validated with the aid of harmonic impedance scans.Steady state quantities between both models are shown to have similar values.The response of both models to a current order step-response and AC faults are compared.This comparison is visually analyzed on a common per-cycle axis and shows agreement.The60Hz model proposed in this paper has performance that is similar to the CBM and is adequate for simulations which require a 60Hz version of the well-known benchmark model.R EFERENCES[1]M.Szechtman,T.Wess,and C.V.Thio,“First benchmark model forHVDC control studies,”Electra,vol.135,no.4,pp.56–73,April1991.[2]——,“A benchmark model for HVDC system studies,”in AC and DCPower Transmission,1991.,International Conference on,Sep1991,pp.374–378.[3]M.Faruque,Y.Zhang,and V.Dinavahi,“Detailed modelingof CIGRE HVDC benchmark system using PSCAD/EMTDC and PSB/SIMULINK,”Power Delivery,IEEE Transactions on,vol.21,no.1, pp.378–387,Jan2006.[4] C.Liu,A.Bose,and P.Tian,“Modeling and analysis of HVDC converterby three-phase dynamic phasor,”Power Delivery,IEEE Transactions on, vol.29,no.1,pp.3–12,Feb2014.[5] C.V.Thio,J.Davies,and K.Kent,“Commutation failures in HVDCtransmission systems,”Power Delivery,IEEE Transactions on,vol.11, no.2,pp.946–957,Apr1996.[6]K.Meah and A.Sadrul Ula,“Simulation study of the CIGRE HVDCbenchmark model with the WSCC nine-bus power system network,”in Power Systems Conference and Exposition,2009.PSCE’09.IEEE/PES, March2009,pp.1–5.[7] E.Rahimi,A.Gole,J.Davies,I.Fernando,and K.Kent,“Commutationfailure analysis in multi-infeed HVDC systems,”Power Delivery,IEEE Transactions on,vol.26,no.1,pp.378–384,Jan2011.[8]X.Chen,A.Gole,M.Han,and C.Liu,“Influence of the MIIF indexon operation of multi-infeed HVDC systems,”in Electrical Power and Energy Conference(EPEC),2011IEEE,Oct2011,pp.216–221. [9] C.Guo,Y.Zhang,A.Gole,and C.Zhao,“Analysis of dual-infeedHVDC with LCC-HVDC and VSC-HVDC,”Power Delivery,IEEE Transactions on,vol.27,no.3,pp.1529–1537,July2012.[10]Y.Liu and Z.Chen,“Aflexible power control method of VSC-HVDClink for the enhancement of effective short-circuit ratio in a hybrid multi-infeed HVDC system,”Power Systems,IEEE Transactions on,vol.28, no.2,pp.1568–1581,May2013.[11]X.Chen,A.Gole,and M.Han,“Analysis of mixed inverter/rectifiermulti-infeed HVDC systems,”Power Delivery,IEEE Transactions on, vol.27,no.3,pp.1565–1573,July2012.[12] A.Daneshpooy,A.Gole,D.Chapman,and J.Davies,“Fuzzy logiccontrol for HVDC transmission,”Power Delivery,IEEE Transactions on,vol.12,no.4,pp.1690–1697,Oct1997.[13]J.Bauman and M.Kazerani,“Commutation failure reduction in HVDCsystems using adaptive fuzzy logic controller,”Power Systems,IEEE Transactions on,vol.22,no.4,pp.1995–2002,Nov2007.[14]Y.Z.Sun,L.Peng,F.Ma,G.J.Li,and P.F.Lv,“Design a fuzzycontroller to minimize the effect of HVDC commutation failure on power system,”Power Systems,IEEE Transactions on,vol.23,no.1, pp.100–107,Feb2008.[15] B.Das,N.Watson,and Y.Liu,“Simulation study of conventional andhybrid HVDC rectifier based on CIGRE benchmark model using PLL-less synchronisation scheme,”in Power Engineering and Optimization Conference(PEOCO),20115th International,June2011,pp.312–317.[16] D.Povh,P.Thepparat,and D.Westermann,“Further development ofHVDC control,”in PowerTech,2011IEEE Trondheim,June2011,pp.1–8.[17]Y.Li, F.Liu,L.Luo, C.Rehtanz,and Y.Cao,“Enhancement ofcommutation reliability of an HVDC inverter by means of an inductive filtering method,”Power Electronics,IEEE Transactions on,vol.28, no.11,pp.4917–4929,Nov2013.[18]X.Jiang and A.Gole,“A frequency scanning method for the identifica-tion of harmonic instabilities in HVDC systems,”Power Delivery,IEEE Transactions on,vol.10,no.4,pp.1875–1881,Oct1995.[19]P.Kundur,Power Sytsem Stability and Control.McGraw-Hill,1994.[20]M.Szechtman et al.,“The CIGRE HVDC benchmark model-a newproposal with revised parameters,”Electra,vol.157,no.2,pp.61–66, December1994.[21]J.Jardini,A.Gole et al.,“TB563:Modelling and simulation studies tobe performed during the lifecycle of HVDC systems,”CIGRE Working Group B4.38,Tech.Rep.,2013.。
数字电路英文版第十三单元
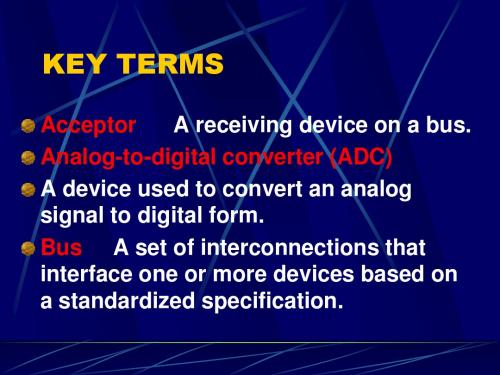
Acceptor A receiving device on a bus. Analog-to-digital converter (ADC) A device used to convert an analog signal to digital form. Bus A set of interconnections that interface one or more devices based on a standardized specification.
High-Z The high-impedance state of a tristate circuit in which the output is effectively disconnected from the rest of the circuit. Interfacing The process of making two or more electronic devices or systems operationally compatible with each other so that they can function properly together.
Peripheral A device or instrument that provides communication with a computer or provides auxiliary services or functions for a computer. Port The physical interface between a computer and a peripheral. SCSI Small computer system interface; an external parallel bus standard.
NEC Digital Single Line (DSL) Analog Single Line (
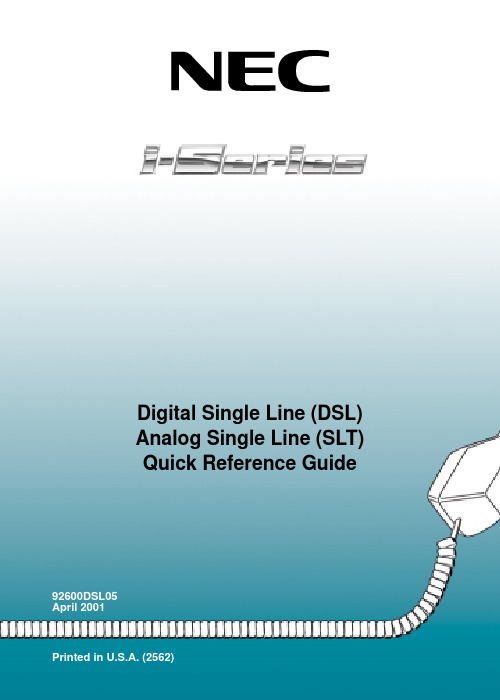
1. (DSL only) If you hear two short beeps: Speak toward your phone.
• You can lift the handset for privacy.
OR
1. If you hear one long ring: Lift handset to speak.
Due to the flexibility built into the system, your Dialing Codes and Feature Capacities may differ from those in this guide. Check with your communications manager and make a note of any differences.
Hold
Use Hold instead of leaving the handset
off-hook:
1. Do not hang up +
+ Do not hang up.
• For DSLs, this puts your outside call
on System Hold. Your co-workers can
OR
2. For Paging, dial or 1-64 for zones.
+ 0 for All Call
OR
2. To call your mailbox, dial
.
If your call doesn’t go through . . .
Camp On and Callback
When you hear sys- 1.
UM10204
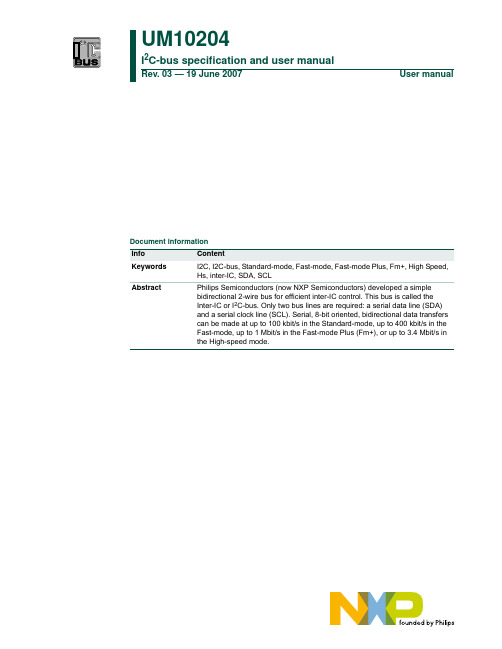
UM10204I2C-bus specification and user manualRev. 03 — 19 June 2007User manualDocument informationInfo ContentKeywords I2C, I2C-bus, Standard-mode, Fast-mode, Fast-mode Plus, Fm+, High Speed, Hs, inter-IC, SDA, SCLAbstract Philips Semiconductors (now NXP Semiconductors) developed a simplebidirectional 2-wire bus for efficient inter-IC control. This bus is called theInter-IC or I2C-bus. Only two bus lines are required: a serial data line (SDA)and a serial clock line (SCL). Serial, 8-bit oriented, bidirectional data transferscan be made at up to 100kbit/s in the Standard-mode, up to 400kbit/s in theFast-mode, up to 1Mbit/s in the Fast-mode Plus (Fm+), or up to 3.4Mbit/s inthe High-speed mode.Contact informationFor additional information, please visit: For sales office addresses, please send an email to: salesaddresses@Revision history Rev Date Description 0320070619Many of today’s applications require longer buses and/or faster speeds. Fast-mode plus wasintroduced to meet this need by increasing drive strength by as much as 10× and increasingthe data rate to 1Mbit/s while maintaining downward compatibility to Fast-mode andStandard-mode speeds and software commands.Modifications:•Re-ordered sections and clarified several requirements •Added description of Fast-mode Plus (Fm+) specifications •Added description of the Device ID Field •Added Bus Clear procedures •Moved level shifting information to a separate application note (AN10441)•Clarified the process of sizing R p •Added limits for t VD;DAT and t VD;ACK 2.12000Version 2.1 of the I 2C-bus specification 2.01998The I 2C-bus has become a de facto world standard that is now implemented in over1000different ICs and licensed to more than 50 companies. Many of today’s applications,however, require higher bus speeds and lower supply voltages. This updated version of theI 2C-bus specification meets those requirements.1.01992Version 1.0 of the I 2C-bus specificationOriginal 1982first release1.IntroductionThe I2C-bus is a de facto world standard that is now implemented in over 1000 differentICs manufactured by more than 50 companies. Additionally, the versatile I2C-bus is usedin a variety of control architectures such as System Management Bus (SMBus), PowerManagement Bus (PMBus), Intelligent Platform Management Interface (IPMI), andAdvanced Telecom Computing Architecture (ATCA).This document will assist device and system designers to understand how the I2C-busworks and implement a working application. Various operating modes are described. Itcontains a comprehensive introduction to the I2C-bus data transfer, handshaking and busarbitration schemes. Detailed sections cover the timing and electrical specifications for theI2C-bus in each of its operating modes.Designers of I2C-compatible chips should use this document as a reference and ensurethat new devices meet all limits specified in this document. Designers of systems thatinclude I2C devices should review this document and also refer to individual componentdata sheets.2.I2C-bus featuresIn consumer electronics, telecommunications and industrial electronics, there are oftenmany similarities between seemingly unrelated designs. For example, nearly everysystem includes:•Some intelligent control, usually a single-chip microcontroller•General-purpose circuits like LCD and LED drivers, remote I/O ports, RAM,EEPROM, real-time clocks or A/D and D/A converters•Application-oriented circuits such as digital tuning and signal processing circuits forradio and video systems, temperature sensors, and smart cardsTo exploit these similarities to the benefit of both systems designers and equipmentmanufacturers, as well as to maximize hardware efficiency and circuit simplicity, PhilipsSemiconductors (now NXP Semiconductors) developed a simple bidirectional 2-wire busfor efficient inter-IC control. This bus is called the Inter IC or I2C-bus. All I2C-buscompatible devices incorporate an on-chip interface which allows them to communicatedirectly with each other via the I2C-bus. This design concept solves the many interfacingproblems encountered when designing digital control circuits.Here are some of the features of the I2C-bus:•Only two bus lines are required; a serial data line (SDA) and a serial clock line (SCL).•Each device connected to the bus is software addressable by a unique address and simple master/slave relationships exist at all times; masters can operate asmaster-transmitters or as master-receivers.•It is a true multi-master bus including collision detection and arbitration to prevent data corruption if two or more masters simultaneously initiate data transfer.•Serial, 8-bit oriented, bidirectional data transfers can be made at up to 100kbit/s inthe Standard-mode, up to 400kbit/s in the Fast-mode, up to 1Mbit/s in Fast-modePlus, or up to 3.4Mbit/s in the High-speed mode.•On-chip filtering rejects spikes on the bus data line to preserve data integrity.•The number of ICs that can be connected to the same bus is limited only by a maximum bus capacitance. More capacitance may be allowed under someconditions. Refer to Section7.2.Figure1 shows an example of I2C-bus applications.2.1Designer benefitsI2C-bus compatible ICs allow a system design to rapidly progress directly from afunctional block diagram to a prototype. Moreover, since they ‘clip’ directly onto theI2C-bus without any additional external interfacing, they allow a prototype system to be modified or upgraded simply by ‘clipping’ or ‘unclipping’ ICs to or from the bus.Here are some of the features of I2C-bus compatible ICs that are particularly attractive to designers:•Functional blocks on the block diagram correspond with the actual ICs; designs proceed rapidly from block diagram to final schematic.•No need to design bus interfaces because the I2C-bus interface is already integrated on-chip.•Integrated addressing and data-transfer protocol allow systems to be completely software-defined.•The same IC types can often be used in many different applications.•Design-time reduces as designers quickly become familiar with the frequently used functional blocks represented by I2C-bus compatible ICs.•ICs can be added to or removed from a system without affecting any other circuits on the bus.•Fault diagnosis and debugging are simple; malfunctions can be immediately traced.•Software development time can be reduced by assembling a library of reusable software modules.In addition to these advantages, the CMOS ICs in the I2C-bus compatible range offerdesigners special features which are particularly attractive for portable equipment and battery-backed systems.They all have:•Extremely low current consumption•High noise immunity•Wide supply voltage range•Wide operating temperature range.2.2Manufacturer benefitsI2C-bus compatible ICs not only assist designers, they also give a wide range of benefits to equipment manufacturers because:•The simple 2-wire serial I2C-bus minimizes interconnections so ICs have fewer pins and there are not so many PCB tracks; result—smaller and less expensive PCBs.•The completely integrated I2C-bus protocol eliminates the need for address decoders and other ‘glue logic’.•The multi-master capability of the I2C-bus allows rapid testing and alignment of end-user equipment via external connections to an assembly-line.•The availability of I2C-bus compatible ICs in a variety of leadless packages reduces space requirements even more.These are just some of the benefits. In addition, I2C-bus compatible ICs increase system design flexibility by allowing simple construction of equipment variants and easyupgrading to keep designs up-to-date. In this way, an entire family of equipment can be developed around a basic model. Upgrades for new equipment, or enhanced-featuremodels (i.e., extended memory, remote control, etc.) can then be produced simply byclipping the appropriate ICs onto the bus. If a larger ROM is needed, it is simply a matter of selecting a microcontroller with a larger ROM from our comprehensive range. As new ICs supersede older ones, it is easy to add new features to equipment or to increase its performance by simply unclipping the outdated IC from the bus and clipping on itssuccessor.2.3IC designer benefitsDesigners of microcontrollers are frequently under pressure to conserve output pins. TheI2C protocol allows connection of a wide variety of peripherals without the need forseparate addressing or chip enable signals. Additionally, a microcontroller that includes anI2C interface will be more successful in the marketplace due to the wide variety of existingperipheral devices available.3.The I2C-bus protocolTwo wires, serial data (SDA) and serial clock (SCL), carry information between thedevices connected to the bus. Each device is recognized by a unique address (whetherit is a microcontroller, LCD driver, memory or keyboard interface) and can operate aseither a transmitter or receiver, depending on the function of the device. An LCD drivermay be only a receiver, whereas a memory can both receive and transmit data. In additionto transmitters and receivers, devices can also be considered as masters or slaves whenperforming data transfers (see Table1). A master is the device which initiates a datatransfer on the bus and generates the clock signals to permit that transfer. At that time,any device addressed is considered a slave.Table 1.Definition of I2C-bus terminologyTerm DescriptionTransmitter the device which sends data to the busReceiver the device which receives data from the busMaster the device which initiates a transfer, generates clock signals andterminates a transferSlave the device addressed by a masterMulti-master more than one master can attempt to control the bus at the same timewithout corrupting the messageArbitration procedure to ensure that, if more than one master simultaneously tries tocontrol the bus, only one is allowed to do so and the winning message isnot corruptedSynchronization procedure to synchronize the clock signals of two or more devicesThe I2C-bus is a multi-master bus. This means that more than one device capable ofcontrolling the bus can be connected to it. As masters are usually microcontrollers, let’sconsider the case of a data transfer between two microcontrollers connected to theI2C-bus (see Figure2).This highlights the master-slave and receiver-transmitter relationships to be found on the I2C-bus. It should be noted that these relationships are not permanent, but only depend on the direction of data transfer at that time. The transfer of data would proceed as follows:1.Suppose microcontroller A wants to send information to microcontroller B:–microcontroller A (master), addresses microcontroller B (slave)–microcontroller A (master-transmitter), sends data to microcontroller B (slave-receiver)–microcontroller A terminates the transfer.2.If microcontroller A wants to receive information from microcontroller B:–microcontroller A (master) addresses microcontroller B (slave)–microcontroller A (master-receiver) receives data from microcontroller B (slave-transmitter)–microcontroller A terminates the transfer.Even in this case, the master (microcontroller A) generates the timing and terminates the transfer.The possibility of connecting more than one microcontroller to the I2C-bus means that more than one master could try to initiate a data transfer at the same time. To avoid the chaos that might ensue from such an event, an arbitration procedure has been developed. This procedure relies on the wired-AND connection of all I2C interfaces to the I2C-bus.If two or more masters try to put information onto the bus, the first to produce a ‘one’ when the other produces a ‘zero’ will lose the arbitration. The clock signals during arbitration are a synchronized combination of the clocks generated by the masters using the wired-AND connection to the SCL line (for more detailed information concerning arbitration see Section3.8).Generation of clock signals on the I2C-bus is always the responsibility of master devices; each master generates its own clock signals when transferring data on the bus. Bus clock signals from a master can only be altered when they are stretched by a slow slave device holding down the clock line or by another master when arbitration occurs.Table2 summarizes the use of mandatory and optional portions of the I2C-busspecification and which system configurations use them.Table 2.Applicability of I2C-bus protocol featuresM = mandatory; O = optional; n/a = not applicable.Feature ConfigurationSingle master Multi-master Slave[1] START condition M M MSTOP condition M M MAcknowledge M M MSynchronization n/a M n/aArbitration n/a M n/aClock stretching O[2]O[2]O7-bit slave address M M M10-bit slave address O O OGeneral Call address O O OSoftware Reset O O OSTART byte n/a O[3]n/aDevice ID n/a n/a O[1]Also refers to a master acting as a slave.[2]Clock stretching is a feature of some slaves. If no slaves in a system can stretch the clock (hold SCL LOW),the master need not be designed to handle this procedure.[3]‘Bit banging’ (software emulation) multi-master systems should consider a START byte. See Section3.15.3.1SDA and SCL signalsBoth SDA and SCL are bidirectional lines, connected to a positive supply voltage via a current-source or pull-up resistor (see Figure3). When the bus is free, both lines areHIGH. The output stages of devices connected to the bus must have an open-drain or open-collector to perform the wired-AND function. Data on the I2C-bus can be transferred at rates of up to 100kbit/s in the Standard-mode, up to 400kbit/s in the Fast-mode, up to 1Mbit/s in Fast-mode Plus, or up to 3.4Mbit/s in the High-speed mode. The number of interfaces connected to the bus is limited by the bus capacitance.For a single master application, the master’s SCL output can be a push-pull driver design provided that there are no devices on the bus which would stretch the clock.3.2SDA and SCL logic levelsDue to the variety of different technology devices (CMOS, NMOS, bipolar) that can be connected to the I2C-bus, the levels of the logical ‘0’ (LOW) and ‘1’ (HIGH) are not fixed and depend on the associated level of V DD. Input reference levels are set as 30% and 70% of V DD; V IL is 0.3V DD and V IH is 0.7V DD. See Figure27, timing diagram. Somelegacy device input levels were fixed at V IL=1.5V and V IH=3.0V, but all new devices require this 30%/70% specification. See Section6 for electrical specifications.3.3Data validityThe data on the SDA line must be stable during the HIGH period of the clock. The HIGH or LOW state of the data line can only change when the clock signal on the SCL line is LOW (see Figure4). One clock pulse is generated for each data bit transferred.3.4START and STOP conditionsAll transactions begin with a START (S) and can be terminated by a STOP (P) (seeFigure5). A HIGH to LOW transition on the SDA line while SCL is HIGH defines a START condition. A LOW to HIGH transition on the SDA line while SCL is HIGH defines a STOP condition.START and STOP conditions are always generated by the master. The bus is considered to be busy after the START condition. The bus is considered to be free again a certain time after the STOP condition. This bus free situation is specified in Section6.The bus stays busy if a repeated START (Sr) is generated instead of a STOP condition. In this respect, the START (S) and repeated START (Sr) conditions are functionally identical.For the remainder of this document, therefore, the S symbol will be used as a generic term to represent both the START and repeated START conditions, unless Sr is particularly relevant.Detection of START and STOP conditions by devices connected to the bus is easy if they incorporate the necessary interfacing hardware. However, microcontrollers with no such interface have to sample the SDA line at least twice per clock period to sense thetransition.3.5Byte formatEvery byte put on the SDA line must be 8bits long. The number of bytes that can betransmitted per transfer is unrestricted. Each byte has to be followed by an Acknowledge bit. Data is transferred with the Most Significant Bit (MSB) first (see Figure6). If a slave cannot receive or transmit another complete byte of data until it has performed some other function, for example servicing an internal interrupt, it can hold the clock line SCL LOW to force the master into a wait state. Data transfer then continues when the slave is ready for another byte of data and releases clock line SCL.3.6Acknowledge (ACK) and Not Acknowledge (NACK)The acknowledge takes place after every byte. The acknowledge bit allows the receiver to signal the transmitter that the byte was successfully received and another byte may be sent. All clock pulses including the acknowledge 9th clock pulse are generated by themaster.The Acknowledge signal is defined as follows: the transmitter releases the SDA lineduring the acknowledge clock pulse so the receiver can pull the SDA line LOW and itremains stable LOW during the HIGH period of this clock pulse (see Figure4). Set-up and hold times (specified in Section6) must also be taken into account.When SDA remains HIGH during this 9th clock pulse, this is defined as the NotAcknowledge signal. The master can then generate either a STOP condition to abort the transfer, or a repeated START condition to start a new transfer. There are five conditions that lead to the generation of a NACK:1.No receiver is present on the bus with the transmitted address so there is no device torespond with an acknowledge.2.The receiver is unable to receive or transmit because it’s performing some real-timefunction and is not ready to start communication with the master.3.During the transfer the receiver gets data or commands that it does not understand.4.During the transfer, the receiver cannot receive any more data bytes.5.A master-receiver needs to signal the end of the transfer to the slave transmitter.3.7Clock synchronizationTwo masters can begin transmitting on an idle bus at the same time and there needs to bea method for deciding which will take control of the bus and complete its transmission.This is done by clock synchronization and arbitration. In single master systems, clocksynchronization and arbitration are not needed.Clock synchronization is performed using the wired-AND connection of I2C interfaces to the SCL line. This means that a HIGH to LOW transition on the SCL line will cause the masters concerned to start counting off their LOW period and, once a master clock has gone LOW, it will hold the SCL line in that state until the clock HIGH state is reached (see Figure7). However, the LOW to HIGH transition of this clock may not change the state of the SCL line if another clock is still within its LOW period. The SCL line will therefore be held LOW by the master with the longest LOW period. Masters with shorter LOW periods enter a HIGH wait-state during this time.When all masters concerned have counted off their LOW period, the clock line will bereleased and go HIGH. There will then be no difference between the master clocks and the state of the SCL line, and all the masters will start counting their HIGH periods. The first master to complete its HIGH period will again pull the SCL line LOW.In this way, a synchronized SCL clock is generated with its LOW period determined by the master with the longest clock LOW period, and its HIGH period determined by the one with the shortest clock HIGH period.3.8ArbitrationArbitration, like synchronization, refers to a portion of the protocol required only if more than one master will be used in the system. Slaves are not involved in the arbitrationprocedure. A master may start a transfer only if the bus is free. Two masters maygenerate a START condition within the minimum hold time (t HD;STA) of the STARTcondition which results in a valid START condition on the bus. Arbitration is then required to determine which master will complete its transmission.Arbitration proceeds bit by bit. During every bit, while SCL is HIGH, each master checks to see if the SDA level matches what it has sent. This process may take many bits. Twomasters can actually complete an entire transaction without error, as long as thetransmissions are identical. The first time a master tries to send a HIGH, but detects that the SDA level is LOW, the master knows that it has lost the arbitration and will turn off its SDA output driver. The other master goes on to complete its transaction.No information is lost during the arbitration process. A master that loses the arbitration can generate clock pulses until the end of the byte in which it loses the arbitration and must restart its transaction when the bus is idle.If a master also incorporates a slave function and it loses arbitration during the addressing stage, it is possible that the winning master is trying to address it. The losing master must therefore switch over immediately to its slave mode.Figure8 shows the arbitration procedure for two masters. Of course, more may be involved depending on how many masters are connected to the bus. The moment there is a difference between the internal data level of the master generating DATA1 and the actual level on the SDA line, the DATA1 output is switched off. This will not affect the data transfer initiated by the winning master.Since control of the I2C-bus is decided solely on the address and data sent by competing masters, there is no central master, nor any order of priority on the bus.There is an undefined condition if the arbitration procedure is still in progress at the moment when one master sends a repeated START or a STOP condition while the other master is still sending data. In other words, the following combinations result in an undefined condition:•Master 1 sends a repeated START condition and master 2 sends a data bit.•Master 1 sends a STOP condition and master 2 sends a data bit.•Master 1 sends a repeated START condition and master 2 sends a STOP condition.3.9Clock stretchingClock stretching pauses a transaction by holding the SCL line LOW. The transactioncannot continue until the line is released HIGH again. Clock stretching is optional and in fact, most slave devices do not include an SCL driver so they are unable to stretch theclock.On the byte level, a device may be able to receive bytes of data at a fast rate, but needs more time to store a received byte or prepare another byte to be transmitted. Slaves can then hold the SCL line LOW after reception and acknowledgment of a byte to force themaster into a wait state until the slave is ready for the next byte transfer in a type ofhandshake procedure (see Figure7).On the bit level, a device such as a microcontroller with or without limited hardware for the I2C-bus, can slow down the bus clock by extending each clock LOW period. The speed of any master is thereby adapted to the internal operating rate of this device.In Hs-mode, this handshake feature can only be used on byte level (see Section5.3.2).3.10The slave address and R/W bitData transfers follow the format shown in Figure9. After the START condition (S), a slave address is sent. This address is 7bits long followed by an eighth bit which is a datadirection bit (R/W)—a ‘zero’ indicates a transmission (WRITE), a ‘one’ indicates a request for data (READ) (refer to Figure10). A data transfer is always terminated by a STOPcondition (P) generated by the master. However, if a master still wishes to communicate on the bus, it can generate a repeated START condition (Sr) and address another slave without first generating a STOP condition. Various combinations of read/write formats are then possible within such a transfer.Possible data transfer formats are:•Master-transmitter transmits to slave-receiver. The transfer direction is not changed (see Figure11). The slave receiver acknowledges each byte.•Master reads slave immediately after first byte (see Figure12). At the moment of the first acknowledge, the master-transmitter becomes a master-receiver and theslave-receiver becomes a slave-transmitter. This first acknowledge is still generated by the slave. Subsequent acknowledges are generated by the master. The STOP condition is generated by the master, which sends a not-acknowledge (A) just prior to the STOP condition.•Combined format (see Figure13). During a change of direction within a transfer, the START condition and the slave address are both repeated, but with the R/W bitreversed. If a master-receiver sends a repeated START condition, it sends anot-acknowledge (A) just prior to the repeated START condition.Notes:bined formats can be used, for example, to control a serial memory. During thefirst data byte, the internal memory location has to be written. After the STARTcondition and slave address is repeated, data can be transferred.2.All decisions on auto-increment or decrement of previously accessed memorylocations, etc., are taken by the designer of the device.3.Each byte is followed by an acknowledgment bit as indicated by the A or A blocks inthe sequence.4.I2C-bus compatible devices must reset their bus logic on receipt of a START orrepeated START condition such that they all anticipate the sending of a slaveaddress, even if these START conditions are not positioned according to the proper format.5.A START condition immediately followed by a STOP condition (void message) is anillegal format. Many devices however are designed to operate properly under this condition.6.Each device connected to the bus is addressable by a unique address. Normally asimple master/slave relationship exists, but it is possible to have multiple identical slaves that can receive and respond simultaneously, for example in a groupbroadcast. This technique works best when using bus switching devices like thePCA9546A where all four channels are on and identical devices are configured at the same time, understanding that it is impossible to determine that each slaveacknowledges, and then turn on one channel at a time to read back each individual device’s configuration to confirm the programming. Refer to individual component data sheets.3.1110-bit addressing10-bit addressing expands the number of possible addresses. Devices with 7-bit and10-bit addresses can be connected to the same I2C-bus, and both 7-bit and 10-bitaddressing can be used in all bus speed modes. Currently, 10-bit addressing is not being widely used.The 10-bit slave address is formed from the first two bytes following a START condition (S) or a repeated START condition (Sr).The first seven bits of the first byte are the combination 11110XX of which the last two bits (XX) are the two Most-Significant Bits (MSBs) of the 10-bit address; the eighth bit of the first byte is the R/W bit that determines the direction of the message.Although there are eight possible combinations of the reserved address bits 1111XXX,only the four combinations 11110XX are used for 10-bit addressing. The remaining four combinations 11111XX are reserved for future I2C-bus enhancements.。
3GPP R4 network architecture

3GPP TS23.002V4.8.0(2003-06)Technical Specification3rd Generation Partnership Project; Technical Specification Group Services and Systems Aspects;Network architecture(Release4)RGLOBAL SYSTEM FORMOBILE COMMUNICATIONSThe present document has been developed within the3rd Generation Partnership Project(3GPP TM)and may be further elaborated for the purposes of3GPP. The present document has not been subject to any approval process by the3GPP Organisational Partners and shall not be implemented.This Specification is provided for future development work within3GPP only.The Organisational Partners accept no liability for any use of this Specification.Specifications and reports for implementation of the3GPP TM system should be obtained via the3GPP Organisational Partners'Publications Offices.KeywordsGSM,UMTS,network,architecture3GPPPostal addressF-06921Sophia Antipolis Cedex-FRANCEOffice address650Route des Lucioles-Sophia AntipolisValbonne-FRANCETel.:+33492944200Fax:+33493654716InternetCopyright NotificationNo part may be reproduced except as authorized by written permission. The copyright and the foregoing restriction extend to reproduction in all media.©2003,3GPP Organizational Partners(ARIB,CWTS,ETSI,T1,TTA,TTC).All rights reserved.ContentsForeword (7)Introduction (7)1Scope (8)2References (8)3Definitions and abbreviations (10)3.1Public Land Mobile Network(PLMN) (11)3.2Core Network(CN)and Access Network(AN) (11)3.3Circuit Switched(CS)and Packet Switched(PS)Domains (11)3.3.1CS Domain (11)3.3.2PS Domain (11)3.4Location register (11)3.5Cell (12)3.6Base Station Controller(BSC)area (12)3.7Radio Network Controller(RNC)area (12)3.8Location Area(LA) (12)3.9Routing Area(RA) (12)3.10MSC area (12)3.11VLR area (12)3.12SGSN area (13)3.13Zones for Regional Subscription (13)3.14Service area (13)3.15Group call area (13)4The basic entities of the mobile system (13)4.1The Core Network(CN)entities (13)4.1.1Entities common to the PS and CS domains (13)4.1.1.1The Home Location Register(HLR) (13)4.1.1.2The Visitor Location Register(VLR) (14)4.1.1.3The Authentication Centre(AuC) (15)4.1.1.4The Equipment Identity Register(EIR) (15)4.1.1.5SMS Gateway MSC(SMS-GMSC) (15)4.1.1.6SMS Interworking MSC (16)4.1.2Entities of the CS domain (16)4.1.2.1The Mobile-services Switching Centre(MSC) (16)4.1.2.1.1MSC Server (16)4.1.2.1.2Circuit Switched-Media Gateway Function(CS-MGW) (16)4.1.2.2The Gateway MSC(GMSC) (17)4.1.2.2.1Gateway MSC Server(GMSC Server) (17)4.1.2.3The Interworking Function(IWF) (17)4.1.3Entities of the PS domain (17)4.1.3.1Serving GPRS Support Node(SGSN) (17)4.1.3.2Gateway GPRS Support Node(GGSN) (18)4.1.3.3Border Gateway(BG) (18)4.2The Access Network(AN)entities (18)4.2.1The Base Station System(BSS) (18)4.2.1.1Base Station Controller(BSC) (19)4.2.1.2Base Transceiver Station(BTS) (19)4.2.2The Radio Network System(RNS) (19)4.2.2.1Radio Network Controller(RNC) (19)4.2.2.2Node B (19)4.3The Mobile Station(MS) (19)4a The specific entities of the mobile system (19)4a.1The Group Call Register(GCR)entity (20)4a.2(void) (20)4a.3The Location Services(LCS)entities (20)4a.3.1Location Services(LCS)entities in RAN (20)4a.3.2Gateway Mobile Location Center(GMLC) (21)4a.4CAMEL entities (21)4a.4.1GSM Service Control Function(gsmSCF) (21)4a.4.2GSM Service Switching Function(gsmSSF) (22)4a.4.3GSM Specialised Resource Function(gsmSRF) (22)4a.4.4GPRS Service Switching Function(gprsSSF) (22)4a.5CBS-specific entities (22)4a.5.1Cell Broadcast Center(CBC) (22)4a.6Number Portability Specific entities (22)4a.6.1IN-based solution:Number Portability Database(NPDB) (22)4a.6.2Signalling Relay-based solution:Mobile Number Portability/Signalling Relay function(MNP-SRF) (22)4a.7Signalling Gateway Function(SGW) (23)5Configuration of a Public Land Mobile Network (23)5.1Basic configuration (23)5.2Configuration of LCS entities (25)5.2.1Configuration of LCS entities for GERAN (25)5.2.2Configuration of LCS entities for UTRAN (25)5.3Configuration of CAMEL entities (26)5.4Configuration of CBS entities (27)5.5Configuration of Signalling Gateway Function (27)6PLMN basic interfaces and reference points (27)6.1Interfaces between Mobile Station and the Fixed Infrastructure (28)6.1.1Interface between Mobile Station and Base Station System(Um-interface) (28)6.1.2Interface between Mobile Station and Radio Netwok System(Uu-interface) (28)6.2Interface between the Core Network and the Access Network (28)6.2.1Interfaces between the CS domain and the Access Network (28)6.2.1.1Interface between the MSC and Base Station System(A-interface) (28)6.2.1.2Interface between the MSC and RNS(Iu_CS interface) (28)6.2.2Interfaces between the PS domain and the Access Network (28)6.2.2.1Interface between SGSN and BSS(Gb-interface) (28)6.2.2.2Interface between SGSN and RNS(Iu_PS-interface) (29)6.3Interfaces internal to the Access Network (29)6.3.1Interface between BSC and BTS(Abis-interface) (29)6.3.2Interface between RNC and Node B(Iub-interface) (29)6.3.3Interface between two RNCs(Iur-interface) (29)6.4Interfaces internal to the Core Network (29)6.4.1Interfaces internal to the CS domain (29)6.4.1.1Interface between the MSC server and its associated VLR(B-interface) (29)6.4.1.2Interface between the HLR and the MSC server(C-interface) (29)6.4.1.3Interface between the HLR and the VLR(D-interface) (30)6.4.1.4Interface between MSC servers(E-interface) (30)6.4.1.5Interface between MSC server and EIR(F-interface) (30)6.4.1.6Interface between VLRs(G-interface) (30)6.4.1.7Reference point(G)MSC server–CS-MGW(Mc Reference Point) (30)6.4.1.8Reference Point MSC Server–GMSC Server(Nc Reference Point) (31)6.4.1.9Reference Point CS-MGW–CS-MGW(Nb Reference Point) (31)6.4.2Interfaces internal to the PS domain (31)6.4.2.1Interface between SGSN and HLR(Gr-interface) (31)6.4.2.2Interface between SGSN and GGSN(Gn-and Gp-interface) (31)6.4.2.3Signalling Path between GGSN and HLR(Gc-interface) (31)6.4.2.4Interface between SGSN and EIR(Gf-interface) (32)6.4.3Interfaces used by CS and PS domains (32)6.4.3.1Interface between MSC/VLR and SGSN(Gs-interface) (32)6.4.3.2Interface between HLR and AuC(H-Interface) (32)6a PLMN specific interfaces (32)6a.1GCR-specific interface (32)6a.1.1Interface between the MSC and its associated GCR(I-interface) (32)6a.2(void) (32)6a.3LCS-specific interfaces (32)6a.3.1LCS interfaces using MAP (32)6a.3.5Interface between BSC and SMLC(Lb-interface) (33)6a.3.6Interface between Peer SMLCs(Lp-interface) (33)6a.3.7Interface between BTS and LMU(Um-interface) (33)6a.3.8Interface between RNS and Stand-Alone LMU,UE(Uu-interface) (33)6a.4CAMEL-specific interfaces (33)6a.4.1GMSC-gsmSSF interface (33)6a.4.2gsmSSF-gsmSCF interface (33)6a.4.3MSC-gsmSSF interface (34)6a.4.4gsmSCF-HLR interface (34)6a.4.5gsmSCF-gsmSRF interface (34)6a.4.6MSC-gsmSCF interface (34)6a.4.7SGSN-gprsSSF interface (34)6a.4.8gprsSSF-gsmSCF interface (34)6a.5CBS-specific interfaces (34)6a.5.1Interface between the CBC and RNS(Iu_BC Interface) (34)6a.6Number portability specific interfaces (35)6a.6.1IN-based solution (35)6a.6.1.1NPDB to MSC interface (35)6a.6.2Signalling Relay-based solution (35)6a.6.2.1GMSC to MNP-SRF interface (35)6a.6.2.2MNP-SRF to HLR interface (35)7Interface to external networks (35)7.1Interface between the fixed networks and the MSC (35)7.2Interface between GGSN and external data networks(Gi-interface) (35)7.3Interface between GMLC and external LCS Client(Le-interface) (35)Annex A(informative):Description for GLR-related entities and interfaces (36)A.1Normative references (36)A.2Definitions related to Gateway Location Register(GLR) (36)A.2.1Gateway Location Register(GLR) (36)A.2.2Intermediate Mobile-services Switching Centre(IM-MSC) (36)A.2.3Intermediate GPRS Serving Node(IM-GSN) (36)A.3The entities of the mobile system (36)A.3.1Gateway Location Register(GLR) (36)A.3.2Intermediate Mobile-services Switching Centre(IM-MSC) (37)A.3.3Intermediate GPRS Serving Node(IM-GSN) (37)A.4Configuration of a Public Land Mobile Network (37)A.4.1Basic configuration with GLR introduction (37)A.5PLMN interfaces (38)A.5.1Interface between the HLR and the GLR(GLa-interface) (38)A.5.2Interface between the VLR and the GLR(GLb-interface) (38)A.5.3Interface between the SGSN and the GLR(GLc-interface) (38)A.5.4Interface between the GLR and the IM_MSC(GLd-interface) (38)A.5.5Interface between the GLR and the IM_GSN(GLe-interface) (38)A.5.6Interface between the SMS-GMSC and the GLR(GLf-interface) (39)A.5.7Interface between the SMS-GMSC and the IM_MSC(GLg-interface) (39)A.5.8Interface between the MSC and the IM_MSC(GLh-interface) (39)A.5.9Interface between the GMLC and the IM_MSC(GLi-interface) (39)A.5.10Interface between the GGSN and the IM_GSN(GLj-interface) (39)A.5.11Interface between the SGSN and the IM_GSN(GLk-interface) (39)Annex B(informative):Change history (40)ForewordThis Technical Specification(TS)has been produced by the3rd Generation Partnership Project(3GPP).The contents of the present document are subject to continuing work within the TSG and may change following formal TSG approval.Should the TSG modify the contents of the present document,it will be re-released by the TSG with an identifying change of release date and an increase in version number as follows:Version x.y.zwhere:x the first digit:1presented to TSG for information;2presented to TSG for approval;3or greater indicates TSG approved document under change control.y the second digit is incremented for all changes of substance,i.e.technical enhancements,corrections,updates, etc.z the third digit is incremented when editorial only changes have been incorporated in the document.IntroductionThis document presents the possible architectures of the Universal Mobile Telecommuncation System(UMTS), covering both UTRAN and GERAN radio access techonologies.Clause3of the document contains the definition of the PLMN entities.Clause4of the document contains the description of the basic entities of the PLMN,and clause4a contains the description of the specific entities of the PLMN.Clause5of the document contains the configuration of the PLMN.Clauses6,6a and7of the document contain the PLMN's basic and specific interfaces and reference points and the PLMN's interfaces towards other networks.1ScopeThis document offers an overview of the PLMN and its architectures and configuration.The configuration and the functional entities of the PLMN and the interfaces between them are described on a general level in order to cope with possible implementations.These descriptions include interfaces between and within the core networks,the access networks,the user equipment,different service platforms,different domains and subsystems,and functional entities within domains and subsystems.This document covers different architectural aspects with varying level of detail.In general,other specifications shall be referred to for further details;these specifications enable the reader to acquire the full understanding of a system or service feature.Note that this document does not cover,or even list,all features of PLMNs.2ReferencesThe following documents contain provisions which,through reference in this text,constitute provisions of the present document.•References are either specific(identified by date of publication,edition number,version number,etc.)or non-specific.•For a specific reference,subsequent revisions do not apply.•For a non-specific reference,the latest version applies.In the case of a reference to a3GPP document(includinga GSM document),a non-specific reference implicitly refers to the latest version of that document in the sameRelease as the present document.[1]GSM01.04:"Digital cellular telecommunications system(Phase2+);Abbreviations andacronyms".[1a]3GPP TR21.905:"3G Vocabulary".[2]3GPP TS22.016:"Digital cellular telecommunications system(Phase2+);International Mobilestation Equipment Identities(IMEI)".[2a]3GPP TS22.060:"Digital cellular telecommunications system(Phase2+);General Packet radio Service(GPRS);Service Description;Stage1".[2b]3GPP TS22.071:"Digital cellular telecommunications system(Phase2+);Location Services (LCS);Service Description;Stage1".[2c]3GPP TS22.078:"Customised Applications for Mobile network Enhanced Logic(CAMEL);Service description,Stage1".[3]3GPP TS23.003:"Digital cellular telecommunications system(Phase2+);Numbering,addressingand identification".[4][void][5]3GPP TS23.008:"Digital cellular telecommunications system(Phase2+);Organisation ofsubscriber data".[6]3GPP TS23.009:"Digital cellular telecommunications system(Phase2+);Handover procedures".[7]3GPP TS23.012:"Digital cellular telecommunications system(Phase2+);Location registrationprocedures".[8]3GPP TS23.041:"Technical realization of Cell Broadcast Service(CBS)".[9](void)[9a]3GPP TS23.060:"Digital cellular telecommunication system(Phase2+);General Packet Radio Service(GPRS);Service Description;Stage2".[10]3GPP TS23.068:"Digital cellular telecommunications system(Phase2+);Voice Group CallService(VGCS)stage2".[10a]GSM03.64:"Digital cellular telecommunication system(Phase2+);Overall Description of the General Packet Radio Service(GPRS)Radio Interface;Stage2".[10b]void[10c]TS23.078:"Customised Applications for Mobile network Enhanced Logic(CAMEL)Phase3-Stage2".[11]ITU-T Recommendation Q.1214(05/1995):"Distributed Functional Plane for Intelligent NetworkCS-1"[11a]3GPP TS23.101:"General UMTS Architecture".[11b]3GPP TS23.110:"Access Stratum(AS):Services and Functions".[12]GSM04.02R98:"Digital cellular telecommunications system(Phase2+);GSM Public LandMobile Network(PLMN)access reference configuration".[13]GSM08.01:"Digital cellular telecommunications system(Phase2+);Base Station System-Mobile-services Switching Centre(BSS-MSC)interface General aspects".[14]GSM08.02:"Digital cellular telecommunications system(Phase2+);Base Station System-Mobile-services Switching Centre(BSS-MSC)interface Interface principles".[14a]3GPP TS25.410:"UTRAN Iu Interface:General Aspects and Principles".[14b]3GPP TS25.41x-series on definition of the Iu interface.[15]GSM08.04:"Digital cellular telecommunications system(Phase1);Base Station System-Mobile-services Switching Centre(BSS-MSC)interface Layer1specification".[16]GSM08.06:"Digital cellular telecommunications system(Phase2+);Signalling transportmechanism specification for the Base Station System-Mobile-services Switching Centre(BSS-MSC)interface".[17]GSM08.08:"Digital cellular telecommunications system(Phase2+);Mobile Switching Centre-Base Station System(MSC-BSS)interface-Layer3specification".[18]3GPP TS28.020:"Digital cellular telecommunications system(Phase2+);Rate adaption on theBase Station System-Mobile-services Switching Centre(BSS-MSC)interface".[19]GSM08.51:"Digital cellular telecommunications system(Phase2+);Base Station Controller-Base Transceiver Station(BSC-BTS)interface-General aspects".[20]GSM08.52:"Digital cellular telecommunications system(Phase2+);Base Station Controller-Base Transceiver Station(BSC-BTS)interface-Interface principles".[21]GSM08.54:"Digital cellular telecommunications system(Phase2+);Base Station Controller(BSC)to Base Transceiver Station(BTS)interface-Layer1structure of physical circuits". [22]GSM08.56:"Digital cellular telecommunications system(Phase2+);Base Station Controller(BSC)to Base Transceiver Station(BTS)-Layer2specification".[23]GSM08.58:"Digital cellular telecommunications system(Phase2+);Base Station Controller(BSC)to Base Transceiver Station(BTS)interface-Layer3specification".[24]GSM08.60:"Digital cellular telecommunications system(Phase2+);Inband control of remotetranscoders and rate adaptors".[25]GSM08.61:"Digital cellular telecommunications system(Phase2+);Inband control of remotetranscoders and rate adaptors(half rate)".[26]3GPP TS29.002:"Digital cellular telecommunications system(Phase2+);Mobile ApplicationPart(MAP)specification".[27]GSM09.03R98:"Digital cellular telecommunications system(Phase2+);Signalling requirementson interworking between the Integrated Services Digital Network(ISDN)or Public SwitchedTelephone Network(PSTN)and the Public Land Mobile Network(PLMN)".[28]3GPP TS29.004:"Digital cellular telecommunications system(Phase2+);Interworking betweenthe Public Land Mobile Network(PLMN)and the Circuit Switched Public Data Network(CSPDN)".[29]3GPP TS29.005:"Digital cellular telecommunications system(Phase2+);Interworking betweenthe Public Land Mobile Network(PLMN)and the Packet Switched Public Data Network(PSPDN)for Packet Assembly/Disassembly facility(PAD)access".[30]3GPP TS29.006:"Digital cellular telecommunications system(Phase2+);Interworking between aPublic Land Mobile Network(PLMN)and a Packet Switched Public Data Network/IntegratedServices Digital Network(PSPDN/ISDN)for the support of packet switched data transmissionservices".[31]3GPP TS29.007:"Digital cellular telecommunications system(Phase2+);General requirementson interworking between the Public Land Mobile Network(PLMN)and the Integrated ServicesDigital Network(ISDN)or Public Switched Telephone Network(PSTN)".[32]3GPP TS29.010:"Digital cellular telecommunications system(Phase2+);Information elementmapping between Mobile Station-Base Station System and BSS-Mobile-services SwitchingCentre(MS-BSS-MSC)-Signalling procedures and the Mobile Application Part(MAP)".[33]3GPP TS29.011:"Digital cellular telecommunications system(Phase2+);Signalling interworkingfor supplementary services".[34]3GPP TR41.001:"GSM Release specifications".[35]3GPP TS43.051:"GERAN Overall Description,Stage2".[36]3GPP TS25.305:“Functional Stage2Description of UE Positioning in UTRAN”[37]3GPP TS43.059:“Functional Stage2Description of Location Services in GERAN”[38]3GPP TS23.271:"Functional Stage2Description of Location Services"[39]3GPP TS49.031:“Location Services(LCS);Base Station System Application Part LCS Extension(BSSAP-LE)”[40]3GPP TS48.031:“Location Services(LCS);Serving Mobile Location Centre-Serving MobileLocation Centre(SMLC-SMLC);SMLCPP specification”[41]3GPP TS44.071:“Location Services(LCS);Mobile radio interface layer3Location Services(LCS)specification”3Definitions and abbreviationsIn addition to the abbreviations given in the remainder of this clause others are listed in GSM01.04and in TR21.905. The definitions of the entities of the mobile system are given in the next subclause.3.1Public Land Mobile Network(PLMN)A Public Land Mobile Network(PLMN)is established and operated by an administration or Recognized Private Operating Agency(RPOA)for the specific purpose of providing land mobile telecommunications service services to the public.A PLMN may be regarded as an extension of a network(e.g.ISDN);it is a collection of MSCs areas within a common numbering plan(e.g.same National Destination Code)and a common routing plan.The MSCs are the functional interfaces between the fixed networks and a PLMN for call set-up.Functionally the PLMNs may be regarded as independent telecommunications entities even though different PLMNs may be interconnected through the ISDN/PSTN and PDNs for forwarding of calls or network information.A similar type of interconnection may exist for the interaction between the MSCs of one PLMN.3.2Core Network(CN)and Access Network(AN)The PLMN infrastructure is logically divided into a Core Network(CN)and an Access Network(AN)infrastructures,as defined in TS23.101and TS23.110.The CN is logically divided into CS domain and PS domain,as defined in next subclause.The AN is called BSS for GSM and RNS for UMTS,as defined in clause"The Access Network".3.3Circuit Switched(CS)and Packet Switched(PS)Domains The CN is constituted of a Circuit Switched(CS)domain and a Packet Switched(PS)domain.These two domains differ by the way they support user traffic,as explained bellow.These two domains are overlapping,i.e.they contain some common entities.A PLMN can implement only one domain or both domains.3.3.1CS DomainThe CS domain refers to the set of all the CN entities offering"CS type of connection"for user traffic as well as all the entities supporting the related signalling.A"CS type of connection"is a connection for which dedicated network resources are allocated at the connection establishment and released at the connection release.The entities specific to the CS domain are:MSC,GMSC,VLR.All the other CN entities defined in clause"4The basic entities of the mobile system"and not defined as PS domain specific entities(see following subclause)are common to the CS and to the PS domains.3.3.2PS DomainThe PS domain refers to the set of all the CN entities offering"PS type of connection"for user traffic as well as all the entities supporting the related signalling.A"PS type of connection"transports the user information using autonomous concatenation of bits called packets:each packet can be routed independently from the previous one.The entities specific to the PS domain are the GPRS specific entities,i.e.SGSN and GGSN.All the other CN entities defined in clause"4The basic entities of the mobile system"and not defined as CS domain specific entities(see previous subclause)are common to the CS and to the PS domains.3.4Location registerTo enable communication to a mobile station the network must know where this mobile station is located.This information is stored in a function named location register.The location register is handled by four different entities.•The Home Location Register(HLR).The Home Location Register(HLR)is the location register to which a mobile subscriber is assigned for record purposes such as subscriber information.•The Visitor Location Register(VLR).The Visitor Location Register(VLR)is the location register for Circuit Switched(CS)services,other than the HLR, used by an MSC to retrieve information for,e.g.handling of calls to or from a roaming mobile station currently located in its area.•The Serving GPRS Support Node(SGSN).The location register function in the SGSN stores subscription information and location information for Packet Switched (PS)services for each subscriber registered in the SGSN.The SGSN is needed only in a PLMN which supports GPRS.-The Gateway GPRS Support Node(GGSN).The location register function in the GGSN stores subscription information and routeing information(needed to tunnel packet data traffic destined for a GPRS MS to the SGSN where the MS is registered)for each subscriber for which the GGSN has at least one PDP context active.The GGSN is needed only in a PLMN which supports GPRS.3.5CellThe cell is an area of radio coverage identified by a Base station identification as defined in GSM23.003.3.6Base Station Controller(BSC)areaThe Base Station Controller(BSC)area is an area of radio coverage consisting of one or more cells controlled by one BSC.The boundaries of a BSC area and a location area are independent;a location area may span the boundary between BSC area and a BSC area may span the boundary between location areas.3.7Radio Network Controller(RNC)areaThe Radio Network Controller(RNC)area is an area of radio coverage consisting of one or more cells controlled by one RNC.The boundaries of a RNC area and a location area are independent;a location area may span the boundary between RNC area and a RNC area may span the boundary between location areas.3.8Location Area(LA)The Location Area(LA)is defined as an area in which a mobile station may move freely without updating the VLR.A location area may include one or several cells.3.9Routing Area(RA)The Routing Area(RA)is defined as an area in which a mobile station,in certain operation modes,may move freely without updating the SGSN.A routing area may include one or several cells.A RA is always contained within a location area.3.10MSC areaThe MSC area is the part of the network covered by an MSC.An MSC area may consist of one or several location areas. An MSC area may also consist of one or several BSC areas.3.11VLR areaThe VLR area is the part of the network controlled by a VLR.A VLR area may consist of one or several MSC areas.3.12SGSN areaThe SGSN area is the part of the network served by an SGSN.An SGSN area may consist of one or several routing areas.An SGSN area may also consist of one or several BSC areas.There need not be a one to one relationship between SGSN area and MSC/VLR area.3.13Zones for Regional SubscriptionA PLMN operator may define a number of regional subscription areas,each of which is a subset of the service area for an unrestricted mobile subscriber.A regional subscription area may be contained within the service area of a single PLMN,or may lie within the service areas of two or more PLMNs.Each regional subscription area consists of one or more zones;each zone is contained within the service area of a PLMN.The definition of a mobile subscriber's regional subscription area is stored within the HLR per National Destination Code(s)(NDC)of a PLMN and is transferred to the VLRs and/or SGSNs of that PLMN.The VLR and/or SGSN evaluates this information to extract the restricted or accessible MSC and/or SGNS areas and location areas to which the mobile subscriber is allowed to roam.The VLR and/or SGNS informs the HLR if an entire MSC and/or SGNS area is restricted.Zones for Regional Subscription and their handling are defined in TS23.003,TS23.008and TS29.002.3.14Service areaThe service area is defined as an area in which a mobile subscriber can be reached by another(mobile or fixed) subscriber without the subscriber's knowledge of the actual location of the mobile station within the area.A service area may consist of several PLMNs.One service area may consist of one country,be a part of a country or include several countries.The location registration system associated with each service area must thus contain a list of all mobile stations located within that service area.3.15Group call areaThe group call area is a predefined area composed of one or a number of cells to which a particular Voice Group Call Service(VGCS)or Voice Broadcast Service(VBS)call is distributed.The composition of a group call area is predefined in the network.The group call area may include cells of more than one MSC area and cells of more than one PLMN.4The basic entities of the mobile systemTo provide the mobile service as it is defined,it is necessary to introduce some specific functions.These functional entities can be implemented in different equipments or gathered.In any case,exchanges of data occur between these entities.4.1The Core Network(CN)entities4.1.1Entities common to the PS and CS domains4.1.1.1The Home Location Register(HLR)This functional entity is a data base in charge of the management of mobile subscribers.A PLMN may contain one or several HLRs:it depends on the number of mobile subscribers,on the capacity of the equipment and on the organisation of the network.The following kinds of information are stored there:-the subscription information;。
ANSI-TIA-EIA-568
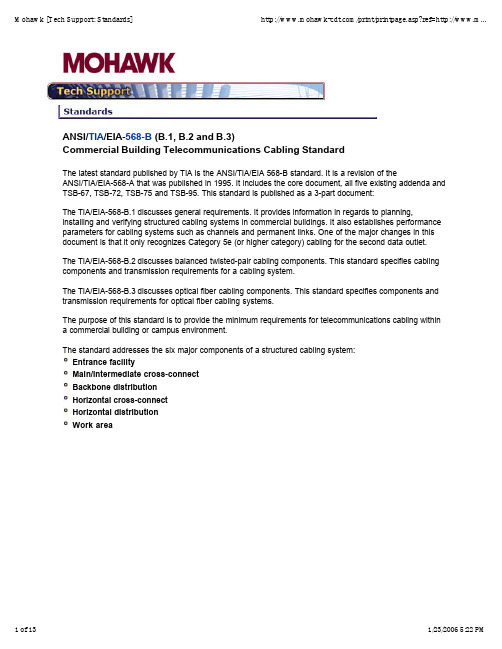
ANSI/TIA /EIA-568-B (B.1, B.2 and B.3)Commercial Building Telecommunications Cabling StandardThe latest standard published by TIA is the ANSI/TIA/EIA 568-B standard. It is a revision of theANSI/TIA/EIA-568-A that was published in 1995. It includes the core document, all five existing addenda and TSB-67, TSB-72, TSB-75 and TSB-95. This standard is published as a 3-part document:The TIA/EIA-568-B.1 discusses general requirements. It provides information in regards to planning,installing and verifying structured cabling systems in commercial buildings. It also establishes performance parameters for cabling systems such as channels and permanent links. One of the major changes in this document is that it only recognizes Category 5e (or higher category) cabling for the second data outlet.The TIA/EIA-568-B.2 discusses balanced twisted-pair cabling components. This standard specifies cabling components and transmission requirements for a cabling system.The TIA/EIA-568-B.3 discusses optical fiber cabling components. This standard specifies components and transmission requirements for optical fiber cabling systems.The purpose of this standard is to provide the minimum requirements for telecommunications cabling within a commercial building or campus environment.The standard addresses the six major components of a structured cabling system:Entrance facilityMain/Intermediate cross-connectBackbone distributionHorizontal cross-connectHorizontal distribution Work areaScope of ANSI/TIA/EIA-568-BENTRANCE FACILITYThe entrance facility contains the cables, connecting hardware, protection devices and other equipment required to connect outside plant facilities to premise cabling. The components within this room may be used for public or private network connections. The demarcation point between service providers and the customer owned premises cabling is typically located in this room.MAIN/INTERMEDIATE CROSS-CONNECTThe backbone distribution topology is based on a hierarchical star topology with no more then two levels of cross-connects, the main cross-connect and the intermediate cross-connect. This will allow the possibility to support a variety of application requirements and will provide a maximum flexibility in the backbone cabling system. The horizontal cross-connect in a TR can be cabled directly to the main cross-connect or to an intermediate cross-connect, then to the main cross-connect.Backbone Distribution in a Hierarchical Star TopologyBACKBONE DISTRIBUTIONThe function of the backbone distribution is to provide interconnection between telecommunications rooms, equipment rooms and entrance facilities to serve the needs of tenants in one or multiple buildings.The components involved in backbone distribution include:Backbone cablesIntermediate and main cross-connectsMechanical terminationsPatch cords or jumpers for backbone-to-backbone connections.General Design GuidelinesPlanning should consider the maximum amount of backbone cable, media additions (optical fiber) and number of connections required during a period spanning from three to ten years.Consider the proximity of metallic cables to possible sources of electromagnetic interference.TopologyThe backbone distribution system is to follow a hierarchical star topology.Each horizontal cross-connect in a TR is cabled to a main cross-connect or an intermediatecross-connect and then a main cross-connect.There cannot be more than two hierarchical levels of cross-connect.At most, one cross-connect can be passed through to go from the horizontal cross-connect to the main cross-connect.Three or fewer cross-connects can be passed through to go from one horizontal cross-connect to a second horizontal cross-connect.Systems designed for non-star configurations (ring, bus or tree) can usually be accommodated by the hierarchical star topology.If special requirements for bus or ring configurations are expected, it is allowable to cable directly between telecommunications closets.This cabling is in addition to the basic star topology.Recognized Backbone Distribution MediaRecognized media may be used individually or in combination.These media are:100 ohm UTP cable50/125 µm optical fiber cable62.5/125 µm optical fiber cableSingle-mode optical fiber cable150 ohm STP-A cable. This media is still a recognized media but is not recommended for new installationsMedia Selection CriteriaThe choice of backbone distribution media will depend on the characteristics of specific applications. Factors to consider in making a selection include:Flexibility with respect to supported servicesRequired useful life of backbone cableSite size and user populationIn-Building and Inter-Building Backbone Cabling DistancesRecommended maximum distances are application and media dependent. It is not assured that all applications will function properly over the specified distances.Maximum Backbone Distribution DistancesMedia TypeHorizontalCross-Connectto MainCross-ConnectHorizontalCross-Connectto IntermediateCross-ConnectMainCross-Connectto IntermediateCross-ConnectUTP800 m(2,624 ft)300 m(984 ft)500 m(1,640 ft)62.5/125 µm or 50/125 µm optical fiber 2,000 m(6,560 ft)300 m(984 ft)1,700 m(5,575 ft)Single-mode optical fiber 3,000 m(9,840 ft)300 m(984 ft)2,700 m(8,855 ft)For high-speed data applications the use of Category 3 or 5e 100 ohm UTP backbone cable shall belimited to a total distance of 90 m (295 ft).The capability of single-mode optical fiber may allow for distance up to 60 km (37 miles), however, this is outside the scope of the standard.Note: These maximum backbone distribution distances are the values found in the ANSI/TIA/EIA-568-B.1 Standard.HORIZONTAL CROSS-CONNECTThe termination of horizontal cable is the primary function of the horizontal cross-connect that is housed in a telecommunications room. Cable of all media types are terminated on compatible connecting hardware. Backbone cable is also terminated on compatible hardware. Connecting hardware, jumper wire and patch cords are collectively referred to as the horizontal cross-connect.Telecommunications Room FunctionsThe primary function is to contain horizontal cable terminations of all recognized types.Recognized types of backbone cable are also terminated here. Cross-connections of horizontal and backbone terminations using jumper wire or patch cords allow for flexibility to extend services to telecommunications outlet/connectors. The intermediate or main cross-connect for portions of the backbone cabling system may also be found in the telecommunications room separate from the horizontalcross-connect.Cross-Connections and InterconnectionsMoves, add-ons or changes are to be completed by performing cross-connects or interconnects.Cross-connects are connections between horizontal cabling and backbone or equipment connecting hardware. Connections made directly between equipment and horizontal cabling are called interconnects.HORIZONTAL DISTRIBUTIONHorizontal distribution is the part of the telecommunications cabling system running from the work area to the horizontal cross-connect in the TR.Horizontal cabling includes:Horizontal distribution cablesTelecommunications outlet/connector in the work areaMechanical termination of the cable mediaPatch cords/jumpers in the TR.Note: May also include a multi-user telecommunications outlet assembly (MUTOA) or a consolidation point (CP).General Design GuidelinesThe horizontal distribution system must satisfy current requirements and should facilitate ongoing maintenance and relocation. Also consider future equipment and service changes.After installation, horizontal cabling is usually less accessible than other cabling.Horizontal cabling is subject to the greatest amount of activity in the building (approx. 90%).Consider the diversity of possible services/applications to be used. Consider the proximity of cables to possible sources of electromagnetic interference.TopologyThe horizontal distribution system must follow a star topology.The telecommunications outlet/connector in the work area is to be directly connected to a horizontal cross-connect in a telecommunications room located on the same floor as the work area.Bridged taps and splices are not permitted.DistancesRegardless of the media type used for horizontal distribution, the maximum distance is 90 m (295 ft).This maximum distance is for the amount of cable required to get from the work area outlet to the horizontal cross-connect in the TR.For each horizontal channel a maximum of 10 m (33 ft) is permitted for work area cords, TR patch cords, jumper wires and equipment cords (inclusive).At the horizontal cross-connect, the maximum length of patch cords/jumpers used to connect horizontal cable to equipment or backbone cable is not to exceed 5 m (16 ft).It is recommended that the maximum length of cord used in the work area should not exceed 5 m (16 ft).Recognized Horizontal Distribution MediaRecognized media may be used individually or in combination.These media are:Four-pair 100 ohm unshielded twisted-pair (UTP) cable50/125 µm optical fiber cable62.5/125 µm optical fiber cable150 ohm STP-A (shielded twisted-pair) cable. This media is still a recognized media but is notrecommended for new installations.Hybrid cables (multiple types of media under a single sheath) may be used in the horizontal distribution system if each recognized cable type meets the transmission requirements and color-code specifications for that cable type.100 ohm UTP cables of mixed categories are not recommended under the same sheath.Crosstalk specifications between cables of a hybrid cable should be met.It must be possible to distinguish hybrid UTP cables from multi-pair UTP backbone cable.Hybrid cable made up of optical fiber and copper conductors may be referred to as composite cable. Media Selection CriteriaEach work area must be equipped with at least two telecommunications outlets/connectors.One outlet may be associated with voice and the other with data.The first outlet shall be a 4-pair 100 ohm UTP cable, Category 3 or higher (Category 5e recommended). The second outlet shall be one of the following media:Four-pair 100 ohm UTP cable Category 5e cableTwo-fiber 50/125 µm optical fiber cableTwo-fiber 62.5/125 µm optical fiber cableWORK AREAWork area components are from the outlet to the work area equipment. It is assumed a maximum of 5 m (16 ft.) is used for the modular cord at the work area. Four-pair UTP cables are terminated in 8-position modular jacks at the work area. The pin/pair assignments are referred to as T568A and T568B.Work Area ComponentsCopper telecommunications outlet/connectorWork area components fall outside the scope of the standard. Work area equipment includes a large variety of equipment. Included are telephones, fax machines, data terminals and computers. Work areas are generally considered to be non-permanent, and are expected to change. Therefore, work areas should be designed to be relatively easy to change.Telecommunications Outlet/ConnectorPin/pair assignment for 100 ohm UTP cable is recommended to follow the T568A or the T568B configuration. These configurations, shown in the illustration below, depict the front view of the telecommunicationsoutlet/connector.It is important to use only one pin/pair configuration (either T568A or T568B) for any given link or channel, and preferably for the entire building.Eight-position jack pin/pair assignmentsOptical fiber telecommunications outlet/connectorThe optical fiber cable used in horizontal cabling shall be terminated at the work area with a duplex optical fiber outlet/connector.The 568SC connector is the recommended connector at the work areaSmall form factor connector type may also be considered.Work Area CordsThe horizontal distribution system assumes a maximum cord length of 5 m (16 ft).Cables and connectors should meet or exceed patch cord recommended requirements.Special AdaptationsIf application specific adaptations such as impedance matching devices are required, they must be external to the telecommunications outlet/connector.Some commonly used adapters include the following:A special cable or adapter when the equipment connector is different from the outlet/connector"Y" adapters to permit two services to run on a single cablePassive adapters used when the horizontal cable type is different from that required by the equipmentActive adapters when connecting devices using different signaling schemesAdapters allowing pair transposition for compatibility purposesTermination resistorsConsider adapter compatibility with premises cabling and equipment. Adapters may have detrimental effects on the transmission performance of the telecommunications cabling system.HORIZONTAL CABLING FOR OPEN OFFICESA horizontal cabling termination point (multi-user telecommunications outlet assembly) and/or intermediate horizontal cabling interconnection point (consolidation point) provide more flexibility in open office layouts with modular furniture, where frequent office rearrangements are performed. Both the multi-user telecommunications outlet assembly and the consolidation point shall be located in a fully accessible, permanent location.Multi-User Telecommunications Outlet AssemblyThe multi-user telecommunications outlet assembly (MUTOA) is a termination point for the horizontal cabling, consisting of several telecommunications outlets in a common location. The modular line cord extends from the MUTOA to the terminal equipment without any additional intermediate connections. This configuration allows the open office plan to change without affecting the horizontal cabling.Multi-User Telecommunications Outlet AssemblyThe following guidelines shall be followed when installing a MUTOA;The MUTOA shall not be installed in a ceilingThe maximum modular cords length shall be 20 m (66 ft.)The modular cord connecting the MUTOA to the terminal equipment shall be labeled on both ends with a unique identifierA MUTOA shall serve a maximum of twelve work areasThe MUTOA shall be marked with the maximum allowable work area cabling (modular cord) length as per the following table:A m (ft.)Bm (ft.)Cm (ft.)Total Channelm (ft.)5 (16)90 (295) 5 (16)100 (328)5 (16)85 (279)9 (30)99 (325)5 (16)80 (262)13 (44)98 (322)5 (16)75 (246)17 (57)97 (319)5 (16)70 (230)22 (72)97 (319)Horizontal and Work Area 24 AWG UTP Cabling LengthsConsolidation PointThe consolidation point (CP) is an interconnection point within the horizontal cabling. The consolidation point performs a "straight-through" intermediate interconnection between the horizontal cabling coming from the horizontal cross-connect and the horizontal cabling going to a MUTOA or the telecommunications outlet in the work area. Cross-connection between these cables is not allowed. A consolidation point may be useful when reconfiguration is frequent. but not so frequent as to require the flexibility of MUTOA.Consolidation PointThe following guidelines shall be followed when installing a consolidation point;Ensure that the total channel distance is 100 meters (330 ft.) or lessIt is recommended that the consolidation point be located at least 15m (49 ft.) from the telecommunications room in order to avoid additional NEXT due to short link resonance of multiple connections in close proximityNo more than one consolidation point and one MUTOA shall be used within the same horizontal runA CP shall serve a maximum of twelve work areasCopper Cabling Transmission PerformanceTransmission performance depends on:Cable characteristicsConnecting hardwarePatch cords and cross-connect wiringNumber of connections (maximum of four)Installation and maintenanceChannel Test ConfigurationChannel testing is used to verify the performance of the overall channel.The channel includes the following components:Up to 90 m (295 ft.) of horizontal cableCable between TR and an optional consolidation point and from the consolidation point to the telecommunications outletWork area cordTelecommunications outlet/connectorCross-Connections in the telecommunications roomPatch cord or jumper wireTelecommunications room equipment cordThe total length of equipment cords, patch cords and jumper wires and work area cords is not to exceed 10m (33 ft.)Channel Test ConfigurationPermanent LinkThe permanent Link test configuration represents the permanently installed cabling. It includes:Up to 90 m (295 ft.) of horizontal cableCable between TR and an optional consolidation point and from the consolidation point to the telecommunications outletA connection at each end of the horizontal cableThe permanent link excludes both the cable portion of the field test instruments cord.Permanent Link Test ConfigurationParameters discussed in the document for channel and permanent links are:Insertion lossNEXT lossPower sum NEXTELFEXT lossPower sum ELFEXTReturn lossPropagation delayDelay skewCategory 5 Cabling TransmissionSince the minimum requirement for copper cabling systems is now Category 5e cabling systems; in the ANSI/TIA/EIA-568-B.1, there is an informative annex that provides cabling transmission performance for Category 5 legacy systems.The important parameters to meet are the Return Loss and the EXFEXT. If an existing Category 5 installation fails either Return Loss or the ELFEXT parameters given in the ANSI/TIA/EIA-568-B.1, annex D, corrective actions should be taken. The document specifies different options to correct the failure. Select the options(s) which is the most appropriate to your solution.Option 1: Replaces the Category 5 patch cords. This will possibly correct ReturnLoss failures.Option 2:If possible, reconfigure the cross-connect as an interconnect.Option 3: Replace the Category 5 transition point or consolidation connector with aCategory 5e transition point or consolidation point connector.Option 4: Replace the Category 5 work area outlet connector with a Category 5ework area outlet connector.Option 5:Replace the Category 5 interconnect with a Category 5e interconnect.Testing should be performed with an enhanced level II (level II-E) field tester or better.Optical Fiber Cabling Transmission PerformanceMinimum recommended performance for a multimode or single-mode optical fiber cabling system are described in this standard.A typical Link segment is found between the telecommunications outlet/connector and the horizontalcross-connect. The link segment includes.Optical fiber cable (length depends on application)ConnectorsSplicesLink ConfigurationThe single performance parameter for optical fiber cabling system is the link attenuation. It is the only parameter that can be affected by the installation and can be tested in the field. Depending on the application, a budget loss is allocated for the link segment.Centralized Optical Fiber CablingThe purpose of the centralized optical fiber cabling system is to provide a fiber-to-the-desk cabling system utilizing centralized electrons versus the traditional method of distributing the electronics to the individual floors.Work area connections are extended to the main cross-connect by utilizing either pull-through cables, an interconnect or a splice in the telecommunications room. Use of an interconnection between the horizontal and backbone cabling provides the greatest flexibility, ease of manageability and can easily be migrated to a cross-connect.The maximum horizontal cabling length is specified at 90m (295 ft.). The distance of horizontal and backbone cabling combined with work area cords, patch cords and equipment cords is not to exceed 300m (984 ft.).Centralized cabling systems shall be located within the same building of the work areas being served. All move and change activity shall be performed at the main cross-connect. Horizontal links may be added and removed in the telecommunications room.When using the pull-through method, the cable is to have a continuous sheath from the work area through the telecommunications room to the centralized cross-connect. The pull-through cable length shall be limited to 300m (984 ft.).When designing a centralized cabling system, provisions shall be made to allow for the migration from pull-through, interconnect or splice to a cross-connect implementation. To facilitate this migration, sufficient space shall be left in the telecommunication room for additional patch panels. In addition, adequate cable slack shall be left in the telecommunications room to allow for the cables to be moved to the cross-connect location.Slack can be stored as either cable or unjacketed fibers. When storing slack, provisions shall be made to ensure bend radius limitations are not violated. Cable slack can be stored within an enclosure or on the wall of the telecommunications room. Protective enclosures shall be used when storing slack fibers.When planning the wall-mount or rack-mount layout, provisions should be made to allow future growth. When sizing backbone cabling, provisions should be made for future horizontal links thereby minimizing the need for additional backbone cables. The backbone fiber count should be capable of supporting present and future networking technologies. Typically two fibers are required for each application connection required at the work area.Labeling of the centralized cabling system shall follow the requirements as specifiedin TIA/EIA-606.To ensure correct fiber polarity, the centralized cabling system shall implement theA-B orientation at the work area and B-A orientation at the centralized cross-connectas specified in ANSI/TIA/EIA-568-B.1Fibers can be joined by either using re-mateable connectors or splices. If connectorsare used, the connector shall meet the specifications as defined inANSI/TIA/EIA-568-B.3. Fibers may be fusion or mechanically spliced, provided therequirements as specified in ANSI/TIA/EIa-568-B.3 are met.New Published Addenda:ANSI/TIA/EIA-568-B.2 addendum 1This addendum provides the Category 6 requirements for channel and permanent links. The Category 6 cabling systems are part of the ANSI/TIA/EIA-568-B core document and follow all the structured cabling requirements, for example, topology, recognized media type, horizontal and backbone design requirements including the work area.ANSI/TIA/EIA-568-B.2 addendum 2This addendum is a revision of sub-clause 4.3.4.8, 4.4, 4.4.1, 4.4.4.9 and 5.4.3 of the ANSI/TIA/EIA-568-B.2 document regarding the NEXT parameter equation for Category 3 cables.ANSI/TIA/EIA-568-B.2 addendum 3This addendum is a revision of clause 1.2.5 regarding additional considerations for insertion loss and return loss pass/fail determination.ANSI/TIA/EIA-568-B.3 addendum 1The purpose of this addendum is to provide additional transmission performance specifications for 50/125 µm optical fiber cable capable of supporting 10 Gb/s serial transmission up to 300m (984 ft.) using 850 nm nominal wavelength lasers. The fiber is called 850 nm Laser-Optimized 50/125 µm multimode optical fiber cable.MOHAWK9 Mohawk Drive Leominster, MA 01453(978) 537-9961 Fax: (978) 537-4358(800) 422-9961 info@Copyright © 1998-2005 Mohawk, a Division of Belden CDT. All rightsreserved. Reproduction in whole or in part in any form or mediumwithout express written permission of Mohawk is prohibited.。
异步切换随机系统的l1增益
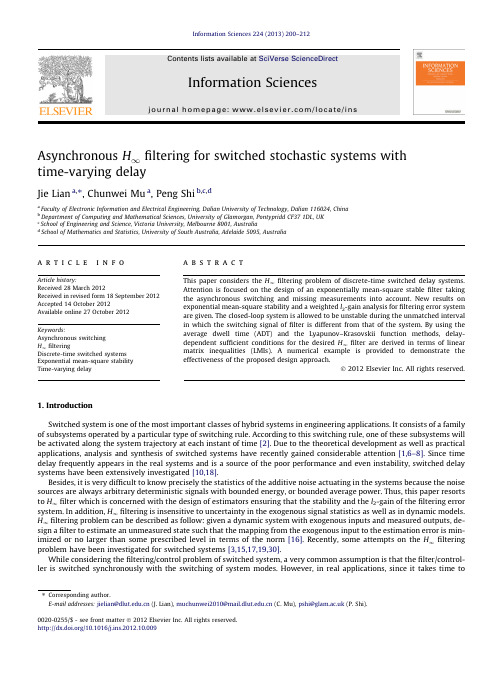
Asynchronous H 1filtering for switched stochastic systems with time-varying delayJie Lian a ,⇑,Chunwei Mu a ,Peng Shi b ,c ,daFaculty of Electronic Information and Electrical Engineering,Dalian University of Technology,Dalian 116024,China bDepartment of Computing and Mathematical Sciences,University of Glamorgan,Pontypridd CF371DL,UK cSchool of Engineering and Science,Victoria University,Melbourne 8001,Australia dSchool of Mathematics and Statistics,University of South Australia,Adelaide 5095,Australiaa r t i c l e i n f o Article history:Received 28March 2012Received in revised form 18September 2012Accepted 14October 2012Available online 27October 2012Keywords:Asynchronous switching H 1filteringDiscrete-time switched systems Exponential mean-square stability Time-varying delaya b s t r a c tThis paper considers the H 1filtering problem of discrete-time switched delay systems.Attention is focused on the design of an exponentially mean-square stable filter taking the asynchronous switching and missing measurements into account.New results on exponential mean-square stability and a weighted l 2-gain analysis for filtering error system are given.The closed-loop system is allowed to be unstable during the unmatched interval in which the switching signal of filter is different from that of the system.By using the average dwell time (ADT)and the Lyapunov–Krasovskii function methods,delay-dependent sufficient conditions for the desired H 1filter are derived in terms of linear matrix inequalities (LMIs).A numerical example is provided to demonstrate the effectiveness of the proposed design approach.Ó2012Elsevier Inc.All rights reserved.1.IntroductionSwitched system is one of the most important classes of hybrid systems in engineering applications.It consists of a family of subsystems operated by a particular type of switching rule.According to this switching rule,one of these subsystems will be activated along the system trajectory at each instant of time [2].Due to the theoretical development as well as practical applications,analysis and synthesis of switched systems have recently gained considerable attention [1,6–8].Since time delay frequently appears in the real systems and is a source of the poor performance and even instability,switched delay systems have been extensively investigated [10,18].Besides,it is very difficult to know precisely the statistics of the additive noise actuating in the systems because the noise sources are always arbitrary deterministic signals with bounded energy,or bounded average power.Thus,this paper resorts to H 1filter which is concerned with the design of estimators ensuring that the stability and the l 2-gain of the filtering error system.In addition,H 1filtering is insensitive to uncertainty in the exogenous signal statistics as well as in dynamic models.H 1filtering problem can be described as follow:given a dynamic system with exogenous inputs and measured outputs,de-sign a filter to estimate an unmeasured state such that the mapping from the exogenous input to the estimation error is min-imized or no larger than some prescribed level in terms of the norm [16].Recently,some attempts on the H 1filtering problem have been investigated for switched systems [3,15,17,19,30].While considering the filtering/control problem of switched system,a very common assumption is that the filter/control-ler is switched synchronously with the switching of system modes.However,in real applications,since it takes time to 0020-0255/$-see front matter Ó2012Elsevier Inc.All rights reserved./10.1016/j.ins.2012.10.009⇑Corresponding author.E-mail addresses:jielian@ (J.Lian),muchunwei2010@ (C.Mu),pshi@ (P.Shi).identify the system modes and activate the matchedfilter/controller,the phenomena of asynchronous switching between system modes andfilter/controller candidates exist.The necessities of considering asynchronous switching for efficient con-troller design have been shown in mechanical or chemical systems[12].Recently,the asynchronous switching problem has been investigated and various methodologies have been developed[21–23,27–29].The stabilization of asynchronous linear system has been included in[22].Stability,l2-gain and asynchronous H1control of discrete-time switched systems are con-sidered in[29].Then,the results are expanded tofiltering problem in[27],which discusses the stability and l2-gain of switched systems.In almost all the works mentioned above,the hypothesis of perfect measurements has been made implicitly.Unfortu-nately,in many practical applications,such a hypothesis does not hold.For example,due to sensor temporal failures or net-work transmission delay/loss,at certain time points,the system measurements only contain noise,which indicate that the real signal is missing.Switched system with missing measurements has received much attention during the past few years. Using binary switching sequence,the missing measurement can be modeled.The binary is specified by a conditional prob-ability distribution taking its values of0and1.Much work has been done on such model[5,9,11,20,24–26].However,when the asynchronously switching and missing measurements happen simultaneously in the systems,stability problem remains open.This paper investigates the asynchronous H1filtering problem for a class of discrete-time switched delay systems with missing measurements.Based on the average dwell time approach,delay-dependent sufficient conditions on exponential mean-square stability and weighted l2-gain analysis are developed for thefiltering error system.Thefiltering error system is allowed to be unstable within a bounded unmatched interval.Then,sufficient conditions for the existence of a desiredfil-ter are established in terms of LMIs.Finally,a numerical example is given to demonstrate the effectiveness of the proposed design approach.The remainder of this paper is organized as follows.The asynchronous H1filtering of switched systems is formulated in Section2.Section3presents our main results.A numerical example is given in Section4,and then we conclude this paper in Section5.Notation:The notations used throughout the paper are standard.The superscript‘T’stands for matrix transposition;R n denotes the n-dimensional Euclidean space;N represents the set of nonnegative integers;the notation P>0means that P is real symmetric and positive definite;l2[0,1)is the space of square-integrable vector functions over[0,1);diag{ÁÁÁ}stands for a block-diagonal matrix;k min(P)(k max(P))denotes the minimum(maximum)eigenvalue of symmetric matrix P;kÁk de-notes the Euclidean norm of a vector and its induced norm of a matrix.In symmetric matrix or long matrix expressions,we use a star(⁄)to represent a term that is induced by symmetry.2.Problem description and preliminariesConsider a class of discrete-time switched delay systems given byx kþ1¼A r x kþA d r x kÀdkþB r x k;z k¼C r x kþC d r x kÀdkþD r x k;~y k ¼C2r x kþC2d r x kÀdkþD2r x k;ð1Þwhere x k2R n is the state vector,x k2R p is the disturbance input which belongs to l2[0,1),z k2R q is the signal to be esti-mated.r is a piecewise constant function of time k called switching signal,which takes its values in thefinite set I¼f1;...;N g,and N>1is the number of subsystems.The positive integer d k denotes the time-varying delay satisfyingd m<d k<d M;ð2Þwhere d m and d M denote the lower and upper bounds of the time-varying delay,respectively.In system(1),~y k is the ideal system output.However,in practical engineering systems,the system output usually is imperfect with probabilistic missing data.Then,the obtained system output can be described byyk¼c kðC2i x kþC2di x kÀd kÞþD2i x k;i2I;ð3Þwhere the stochastic variable c k obeys Bernoulli distributed white sequence specified by the following probabilities:Prob f c k¼1g¼E f c k g¼p;ð4ÞProb f c k¼0g¼1ÀE f c k g¼1Àp;ð5Þwith a known constant p>0.Obviously,for a stochastic variable c k,we have the mean value E{c k}=p and variance q2=p(1Àp).Next,we are interested in designing a full-orderfilter described by^xkþ1¼A ci^x kþB ci y k;^zk¼C ci^x kþD ci y k;ð6Þwhere^x k2R n is the state estimation;^z k2R q is an estimation for z k;A ci,B ci,C ci and D ci are matrices to be determined.J.Lian et al./Information Sciences224(2013)200–212201Here,when the subsystem and its corresponding filter are active at the same time,such running time interval is called matched interval.On the contrary,it is called unmatched interval if they are not active simultaneously.For example,it is assumed that the subsystem is activated at the switching instant k l ;8l 2N .Since the real switching time of filter exceeds or lags behind that of the system,the switching instants of the filter is k l þT ðk l þ1;k l Þ;8l 2N ,where T ðk l þ1;k l Þrepresents the time length during which the switching signal of filter are different from that of the system in interval [k l ,k l +1).So we can get,when k 2½k l ;k l þT ðk l þ1;k l ÞÞ,the switching signal of system is different from that of the filter;when k 2½k l þT ðk l þ1;k l Þ;k l þ1Þ,the switching signal of system is the same with that of the filter.That is ½k l ;k l þT ðk l þ1;k l ÞÞand ½k l þT ðk l þ1;k l Þ;k l þ1Þare the un-matched and matched intervals respectively.Therefore,from (1)and (6),we get the filtering error system as follows:~x k þ1¼A i ~x k þA di H ~x k Àd k þB i x k ;~z k ¼C i ~x k þC di H ~x k Àd k þD i x k ;8k 2½k l ;k l þT ðk l þ1;k l ÞÞ~x k þ1¼e A i ~x k þe A di H ~x k Àd k þe B i x k ;~z k ¼e C i ~x k þe Cdi H ~x k Àd k þe D i x k ;8k 2½k l þT ðk l þ1;k l Þ;k l þ1Þ8>>>>>>><>>>>>>>:ð7Þwhere~x k ¼x T k^x T k ÂÃT;~z k ¼z k À^z k ;H ¼I 0½ ;A i ¼A ic k B cj C 2i A cj"#;e A i ¼A i0c k B ci C 2i A ci"#;A di ¼A dic k B cj C 2di "#;e Adi ¼A dic k B ci C 2di"#;B i ¼B i B cj D 2i"#;e B i ¼B i B ci D 2i"#C i ¼C i Àc kD cj C 2i ÀC cj ½ ;C di ¼C di Àc kD cj C 2di ;e C i ¼C i Àc kD ci C 2i ÀC ci ½ ;e C di ¼C di Àc kD ci C 2di ;i ¼D i ÀD cj D 2i ;e Di ¼D i ÀD ci D 2i ;For convenience,we denote1i ¼A i 0pB cjC 2iA cj"#;2i ¼00B cj C 2i"#;1di ¼A di pB cj C 2di"#;2di ¼0B cj C 2di"#;1i ¼C i ÀpD cj C 2i ÀC cj ÂÃ;2i ¼D cj C 2i 0½ ;1di ¼C di ÀpD cj C 2di ;2di ¼D cj C 2di ;e A1i ¼A i 0pB ci C 2iA ci"#;e A2i ¼00B ci C 2i0"#;e A1di ¼A di pB ci C 2di"#;e A2di ¼0B ci C 2di"#;e C 1i ¼C i ÀpD ciC 2i ÀC ci ½ ;e C2i ¼D ci C 2i 0½ ;e C 1di ¼C di ÀpD ci C 2di ;e C2di ¼D ci C 2di :We give the following definitions,which will play important roles in deriving our main results subsequently.Definition 1.[6]For any T 1>T 2>0,let N (T 1,T 2)be the switching number of r over [T 2,T 1).If N (T 1,T 2)6N 0+(T 1ÀT 2)/s a holdfor N 0P 0and s a >0,then N 0and s a are called chatter bound and average dwell time,respectively.As commonly used in the literature,we choose N 0=0.Definition 2.[25]Consider the filtering error system (7),suppose that there exist constants c >0,d 2(0,1)and f >1such thatE fk x k k 2g 6cd k E fk x k 0k 2g and P 1k ¼k 0f Àk E ~z T k ~z k ÈÉ<c 2P 1k ¼k 0x T k x k hold,then the filtering error system is said to be exponentiallymean-square stable with x k =0under switching signal r and has a weighted l 2-gain no greater than c .3.Main results3.1.Stability and H 1performance analysisIn this section,delay-dependent sufficient conditions on exponential mean-square stability with a weighted l 2-gain are derived for the filtering error system (7)via the average dwell time approach.202J.Lian et al./Information Sciences 224(2013)200–212Theorem 1.Given scalars 0<a <1,b >0and c >0,the filtering error system (7)is exponentially mean-square stable with aweighted l 2-gain c s ¼c ffiffiffiffiffiffiffiffiffiffiffiffiffiffiffiffiffiffiffiffiffiffiffiffiffiffiffiffiffiffiffiffiffiffiffiffiffiffiffiffiffiffiffiffiffiffiffiffiffiffiffiffiffiffiffiffiffiffiffiffiffiffiffiffiffiffiffiffiffiffiffiffiffiffiffiffiffiffiffiffiffiffiffiffiffiffiffiffiffiffiffiffiffiffiffiffiffiffiffiffiffiffiffiffiffiffiffiffiffiffiffip 1h T max ð1À~a p 1=s a 1Þ=ð1À~a p 1=s a 1Þð1À~a h T max =s a p 1=s a1Þq under ADT switching signals r ,if there exist symmetric and positive definite matrices P i ,Q i ,R bi ,Z ci ,and matrices X i ;M ci ;N ci ;b ¼1;2;c ¼1;2;3;i 2I ,such that thefollowing inequalities holdw 1À~a P i H T M T 2i þH T N T2i H T M T 3i þH T N T3i e N 11e N1ÃÀ~a d M Q i 0e N 12e N 2ÃÃÀc 2I e N 13e N 3ÃÃÃe N 40ÃÃÃÃe N 52666666666437777777775<0;ð8Þw 1À bP i H T M T 2i þH T N T2iH T M T 3i þH T N T3iN 11N 1ÃÀ b d m Q i 0N 12N 2ÃÃÀc 2IN 13N 3ÃÃÃN 40ÃÃÃÃN 52666666666437777777775<0;ð9Þwheree N1c ¼ÀN ci ÀM ci s 11M ci s 12N ci ½ ;e N 1¼e C T 1i q e C T 2i e A T 1i X T iq e A T 2i X T i H T ðA T iÀI ÞZ 3i hi;e N 2¼e C T 1di q e C T 2die A T 1di X T iq e A T 2di X T iA T di Z 3ihi ;e N 3¼e D T i 0e B T iX T i 0B T i Z 3ihi;e N 4¼diag À~a d m R 1i À~a d M R 2i Às 11Z 1i Às 12Z 2i ÈÉ;e N 5¼diag ÀI ÀI ~w 2~w 2ÀZ 3i ÈÉ;N 1c ¼ÀN ci ÀM ci s 21M ci s 22N ci ½ ;N 1¼T 1iT 2i T 1iXTj T 2iXTjHTðA T iÀI ÞZ 3i hi;N 2¼C T 1di qC T 2di A T 1di X TjqA T 2di X TjA T di Z 3i hi;N 3¼D T i0B T i X Tj0B T i Z 3i hi;N 4¼diag À bd m R 1i À b d M R 2i Às 21Z 1i Às 22Z 2i ÈÉ;N 5¼diag ÀI ÀI w2 w 2ÀZ 3i ÈÉ;w 1¼H T ðs Q i þR 1i þR 2i ÞH þM 1i H þH T M T 1i þN 1i H þH T N T1i ;~w 2¼P i ÀX T iÀX i ; w 2¼P i ÀX T jÀX j ;Z 3i ¼d M Z 1i þd m Z 2i ;s 11¼ð~aÀd M À1Þ=a ;s 12¼ð~a Àd m À1Þ=a ;s 21¼ð1À bÀd M Þ=b ;s 22¼ð1À b Àd m Þ=b ;s ¼d M Àd m þ1; b ¼1þb ;~a ¼1Àa ;and the average dwell times a >s Ãa ¼ÀðT max ln h þln p 1p 2Þ=ln ~að10Þwhere h ¼ b=~a ;p 1¼max i 2I f j 2i =j 4i g ;j 4i ¼k min ðP i Þþd m s k min ðQ i Þþd m k min ðR 1i Þþd M k min ðR 2i Þp 2¼max i ;j 2I ;i –jf j 1i =j 3jg ;j 3i ¼k min ðP i Þþd m s k min ðQ i Þþd m ~ad m k min ðR 1i Þþd M ~a d M k min ðR 2i Þ;j 1i ¼k max ðP i Þþd M s b d M k max ðQ i Þþd M b d M k max ðR 2i Þþd 2M b d M k max ðZ 1i Þþd 2mb d m k max ðZ 2i Þ;j 2i ¼k max ðP i Þþd M s k max ðQ i Þþd M k max ðR 2i Þþd 2M k max ðZ 1i Þþd 2m k max ðZ 2i Þ;T max ,max 8l 2NT ðk l þ1Àk l Þ:Proof.Denote g l =x l +1Àx l and choose the following Lyapunov–Krasovskii functional asV i ðk Þ¼V 1i ðk ÞþV 2i ðk ÞþV 3i ðk ÞþV 4i ðk Þ;8k 2½k l ;k l þT ðk l þ1;k l ÞÞe V i ðk Þ¼e V1i ðk Þþe V 2i ðk Þþe V 3i ðk Þþe V 4i ðk Þ;8k 2½k l þT ðk l þ1;k lÞ;k l þ1Þ8<:ð11ÞJ.Lian et al./Information Sciences 224(2013)200–212203where1i ðk Þ¼e V 1i ðk Þ¼~x T k P i ~x k ;2i ðk Þ¼X k À1l ¼k Àd k b k Àl À1x T l Q i x l þXk Àd m j ¼k Àd M þ1Xk À1l ¼j b k Àl À1x T lQ i x l ;V 3i ðk Þ¼X k À1l ¼k Àd mb k Àl À1x T lR 1i x l þX k À1l ¼k Àd Mb k Àl À1x T lR 2i x l ;4i ðk Þ¼X k À1j ¼k Àd M X k À1l ¼jb k Àl À1g T l Z 1i g l þXk À1j ¼k Àd m X k À1l ¼j b k Àl À1g T lZ 2i g l ;e V 2i ðk Þ¼X k À1l ¼k Àd k ~a k Àl À1x T l Q i x l þXk Àd m j ¼k Àd M þ1Xk À1l ¼j ~a k Àl À1x T l Q i x l ;e V 3i ðk Þ¼Xk À1l ¼k Àd m ~a k Àl À1x T l R 1i x l þX k À1l ¼k Àd M~a k Àl À1x T l R 2i x l ;e V4i ðk Þ¼X k À1j ¼k Àd M X k À1l ¼j~ak Àl À1gTl Z 1ig l þXk À1j ¼k Àd m X k À1l ¼j~a k Àl À1g T l Z 2i g l :Denote D e Vi ðk Þ¼e V i ðk þ1ÞÀe V i ðk Þ.The subsequent proof is divided into two parts according the matched and unmatched intervals.When k 2½k l þT ðk l þ1;k l Þ;k l þ1Þ,the system and filter activate in the matched intervals.Then we can getE f D e V 1i ðk Þþa e V 1i ðk Þg ¼E ~x T k þ1P i ~x k þ1À~a ~x T kP i ~x k ÈÉ:ð12ÞOwing toE X k l ¼k þ1Àd k þ1~a k Àl x T l Q i x l 8<:9=;6E X k l ¼k þ1Àd k~a k Àl x T l Q i x l þXk Àd m l ¼k þ1ÀdM~a k Àl x T l Q i x l 8<:9=;;Thus,we can getE f D e V2i ðk Þþa e V 2i ðk Þg 6E s x T k Q i x k À~a d k x T k Àd k Q i x k Àd k þX k Àd m l ¼k þ1Àd M~a k Àl x T l Q i x l ÀX k Àd m l ¼k þ1Àd M~a k Àl x T l Q i x l()¼E s x T k Q i x k À~a d M x T k Àd kQ i x k Àd k no:ð13ÞSimilarlyE f D e V 3i ðk Þþa e V 3i ðk Þg ¼E x T k ðR 1i þR 2i Þx k À~a d m x T k Àd m R 1i x k Àd m À~a d M x T k Àd M R 2i x k Àd M n o:ð14ÞE f D e V 4i ðk Þþa e V 4i ðk Þg ¼E d M g T k Z 1i g k þd m g T kZ 2i g k ÀX k À1l ¼k Àd M~a k Àl g T l Z 1i g l ÀX k À1l ¼k Àd m~a k Àl g T l Z 2i g l ():ð15ÞOn the other hand,by means of the Newton–Leibniz formula,it gives rise tox k Àx k Àd M ÀX k À1l ¼k Àd Mg l ¼0;x k Àx k Àd m ÀX k À1l ¼k Àd mg l ¼0:Then,we have2n T k M i x k Àx k Àd M ÀX k À1l ¼k Àd Mg l "#¼0;2n T k N i x k Àx k Àd m ÀX k À1l ¼k Àd mg l "#¼0;ð16Þwheren k ¼~x T kx T k Àd k x T k x T k Àd m x T k Àd Mhi T;M i ¼M T1iM T 2i M T 3i00ÂÃT;N i ¼N T1iN T 2i N T 3i00ÂÃT:204J.Lian et al./Information Sciences 224(2013)200–212From (12)–(16),it is obtained thatE D e V i ðk Þþa e V i ðk Þþ~z T k ~z k Àc 2x T k x k n o ¼E D e V i ðk Þþa e V i ðk Þþ~z T k ~z k Àc 2x T k x k þ2n T k M i x k Àx k Àd M ÀX k À1l ¼k Àd Mg l "#(þ2n T k N i x k Àx k Àd m ÀX k À1l ¼k Àdmg l"#)6E n T k ðU i þs 11M i Z À11i M T i þs 12N i Z À12i N T i Þn k þ~z T k ~z k þ~x T k þ1P i ~x k þ1þg T k Z 3i g knÀX k À1l ¼k Àd M n T k M i þ~a k Àl g T l Z 1iÂÃ~a l Àk Z À11i M T i n k þ~a k Àl Z 1i g l h i ÀX k À1l ¼k Àd mn T k N i þ~a k Àl g T l Z 2i ÂÃ~a l Àk Z À12i N T i n k þ~ak Àl Z 2i g l hi );ð17ÞwhereU i ¼w 1À~a P i H T M T 2i þH T N T2iH T M T 3i þH T N T3iÀN 1i ÀM 1iÃÀ~ad M Q i 0ÀN 2i ÀM 2i ÃÃÀc 2I ÀN 3iÀM 3i ÃÃÃÀ~a d m R 1i 0ÃÃÃÃÀ~ad M R 2i 2666666437777775:In virtue of Z 1i >0and Z 2i >0,the last two terms of (17)are all non-positive.By Schur complement,we haveE f D e Vi ðk Þþa e V i ðk Þg 60;ð18ÞE f D e Vi ðk Þþa e V i ðk Þþ~z T k~z k Àc 2x T kx k g 60:ð19Þif the following inequality holdsw 1À~a P i H T M T 2i þH T N T2i H T M T 3i þH T N T3i e N 11e H1ÃÀ~a d M Q i 0e N 12e H 2ÃÃÀc 2I e N 13e H 3ÃÃÃe N 40ÃÃÃÃe H 4266666664377777775<0;ð20Þwheref H 1¼e C T 1i q e C T 2i e A T 1i q e A T 2iHTðA T iÀI ÞZ 3ihi;f H 3¼e D T i0e B T iB T i Z 3ihi;f H 2¼e C T 1di q e C T 2die A T 1di q e A T 2diA T di Z 3i hi ;f H 4¼diag ÀIÀIÀP À1iÀP À1iÀZ 3i ÈÉ:From the fact ðP i ÀX i ÞP À1i ðP i ÀX i ÞT >0,we have the following inequalities:ÀX i P À1i X T i <P i ÀX i ÀX Ti .Then pre-and post-multiplying (20)by diag I I I I I I I I I X i X i I f g and diag I I I I I I I I I X T i X Ti I ÈÉrespectively,we can get (8).This means that if (8)holds,(20)is true.On the other hand,when k 2½k l ;k l þT ðk l þ1;k l ÞÞ,the subsystem and filter are active in the unmatched interval.Following the similar proof line of stability of systems (7)in the matched intervals,from (9),we haveE f D V i ðk ÞÀb V i ðk Þg 60:ð21ÞE D i ðk ÞÀb i ðk Þþ~z T k ~z k Àc 2x T k x k ÈÉ60:ð22ÞFrom (11),it is obtained thate V r k lðk l þT ðk l þ1;k l ÞÞ6p 1r k lðk l þT ðk l þ1;k lÞÞ:ð23ÞV r k lðk l Þ6p 2e V r kl À1ðk l Þ:ð24ÞCombining with (18),(21),(23)and (24)yieldsE f e V r k ðx k Þg 6E ~a ðk Àk l ÀT ðk ;k l ÞÞe V r k l ðx k l þT ðk ;k l ÞÞn o 6E ~a ðk Àk l ÀT ðk ;k l ÞÞp 1V r k l ðx k l þT ðk ;k l ÞÞn o 6E ~a ðk Àk l Þh T ðk ;k l Þp 1V r k l ðx k l Þn o6E ~a ðk Àk l Þh T ðk ;k l Þp 1p 2e V r k l À1ðx k l Þn o 6E ~a ðk Àk l À1Þh T ðk ;k l À1Þðp 1p 2Þ2e V r k l À2ðx k l À1Þn o6ÁÁÁ6E ~aðk Àk 0Þh ðN r ðk ;k 0Þþ1ÞT max ðp 1p 2ÞN r ðk ;k 0Þp 1V r k 0ðx k 0Þn o 6E p 1h T max ~a h T max =s a ðp 1p 2Þ1=s a k Àk 0V r k 0ðx k 0Þ&':ð25ÞJ.Lian et al./Information Sciences 224(2013)200–212205Thenmin i2I j3i E fk xkk2g6E f V iðkÞg6maxi2Ij1i p1h T max~a h T max=s aðp1p2Þ1=s akÀk0E fk x kk2g:ð26ÞFrom(10),it is obtained~a h T max=s aðp1p2Þ1=s a<1.Therefore,according to Definition2,thefiltering error system(7)is expo-nentially mean-square stable.Next,we will analyze the H1performance of thefiltering error system(7).From(19)and22,23,24,we can getE f e V r kl ðx kÞg6E~a kÀk lÀTðk;k lÞe V r klðx klþTðk;klÞÞÀX kÀ1s¼k lþTðk;klÞ~a kÀsÀ1C s 8<:9=;6E p1~a kÀk lÀTðk;k lÞrk lðx klþTðk;klÞÞÀX kÀ1s¼k lþTðk;klÞ~a kÀsÀ1C s8 < :9 = ;6E~a kÀk l h Tðk;k lÞp1r kl ðx klÞÀXk lþTðk;klÞÀ1s¼k l~a kÀsÀ1h k lþTðk;k lÞÀsÀ1p1C sÀX kÀ1s¼k lþTðk;klÞ~a kÀsÀ1C s8 < :9 = ;6E~a kÀk l h Tðk;k lÞp1p2e V r klÀ1ðx klÞÀXk lþTðk;klÞÀ1s¼k l~a kÀsÀ1h k lþTðk;k lÞÀsÀ1p1C sÀX kÀ1s¼k lþTðk;klÞ~a kÀsÀ1C s8 < :9 = ;:where C s¼~z Ts ~zsÀc2x T s x s.FurthermoreE f e V r kl ðx kÞg6E~a kÀk0h Tðk;k0Þðp1p2ÞNðk;k0Þp1V rk0ðx kÞnÀXk0þTðk1;k0ÞÀ1 s¼k0~a kÀsÀ1h k0þTðk;k0ÞÀsÀ1ðp1p2ÞNðk;k0Þp1C sÀXk1À1s¼k0þTðk1;k0Þ~a kÀsÀ1h Tðk;k1Þðp1p2ÞNðk;k0ÞC sÀÁÁÁÀXk lÀ1þTðkl;k lÀ1ÞÀ1s¼k lÀ1~a kÀsÀ1h k lÀ1þTðk;k lÀ1ÞÀsÀ1p21p2C sÀX k lÀ1s¼k lÀ1þTðkl;k lÀ1Þ~a kÀsÀ1h Tðk;k lÞp1p2C sÀXk lþTðk;klÞÀ1 s¼k l ~a kÀsÀ1h k lþTðk;k lÞÀsÀ1p1C sÀX kÀ1s¼k lþTðk;klÞ~a kÀsÀ1C s9=;:Under the zero-initial condition x(k0)=0,we know thatEXk0þTðk1;k0ÞÀ1s¼k0~a kÀsÀ1h k0þTðk;k0ÞÀsÀ1ðp1p2ÞNðk;k0Þp1z Tsz s8<:þXk1À1s¼k0þTðk1;k0Þ~a kÀsÀ1h Tðk;k1Þðp1p2ÞNðk;k0ÞÂz Tsz sþÁÁÁþXk lÀ1þTðkl;k lÀ1ÞÀ1s¼k lÀ1~a kÀsÀ1h k lÀ1þTðk;k lÀ1ÞÀsÀ1p21p2z Tsz sþX k lÀ1s¼k lÀ1þTðkl;k lÀ1Þ~a kÀsÀ1h Tðk;k lÞp1p2Âz Tsz sþXk lþTðk;klÞÀ1 s¼k l ~a kÀsÀ1h k lþTðk;k lÞÀsÀ1p1z Tsz sÀX kÀ1s¼k lþTðk;klÞ~a kÀsÀ1z Tsz s9=;6Xk0þTðk1;k0ÞÀ1s¼k0~a kÀsÀ1h k0þTðk;k0ÞÀsÀ1ðp1p2ÞNðk;k0Þp1c2x T s x sþXk1À1s¼k0þTðk1;k0Þ~a kÀsÀ1h Tðk;k1Þðp1p2ÞNðk;k0ÞÂc2x T s x sþÁÁÁþXk lÀ1þTðkl;k lÀ1ÞÀ1s¼k lÀ1~a kÀsÀ1h k lÀ1þTðk;k lÀ1ÞÀsÀ1p21p2c2x Tsx sþX k lÀ1s¼k lÀ1þTðkl;k lÀ1Þ~a kÀsÀ1p1p2Âh Tðk;k lÞc2x T s x sþXk lþTðk;klÞÀ1s¼k l~a kÀsÀ1h k lþTðk;k lÞÀsÀ1p1c2x Tsx sÀX kÀ1s¼k lþTðk;klÞ~a kÀsÀ1c2x Tsx s:206J.Lian et al./Information Sciences224(2013)200–212。
- 1、下载文档前请自行甄别文档内容的完整性,平台不提供额外的编辑、内容补充、找答案等附加服务。
- 2、"仅部分预览"的文档,不可在线预览部分如存在完整性等问题,可反馈申请退款(可完整预览的文档不适用该条件!)。
- 3、如文档侵犯您的权益,请联系客服反馈,我们会尽快为您处理(人工客服工作时间:9:00-18:30)。
BUS AND RING SYSTEM INTERCONNECTIONS FOR DATAACQUISITION AND CONTROLV.I. Vinogradov, INR RAN, RF.AbstractThe traditional bus systems based on 8/16 bit microcomputer- and register-oriented interfaces for data acquisition and control are analyzed. Bus- and nonbus-type system and network interconnections are compared. The possible transition from traditional bus to indirect point-to-point interconnections for control systems is discussed. The low interaction-rate nodes of message-passing systems can be exchanged for high-rate ones in operating control networks, with direct control access to distributed equipment based on shared distributed memory subsystems and a new generation microprocessors (µP).Advanced distributed-memory data acquisition and control systems based on a new generation 16/32-bit general purpose microprocessors and Digital Signal Processors (DSP) with RAMLINK-type (SCI-like) distributed memory interconnections are proposed and discussed. Advanced distributed µP systems are proposed and analyzed on the basis of a new generation of DSP and distributed memory.The new international standards for distributed data processing and memory interconnections are discussed. But for control systems an international standard for fieldbuses has not been selected after more than 20 years, though there are some national standards developments. In this case register-oriented bus interfaces on traditional message-passing networks can be changed to distributed shared-memory direct-access interconnections.Some new decisions for fieldbus and instrumentation input-output subsystems can be based on bus and ring interconnections, which must be developed according to measurement, acquisition and control (MAC) requirements, using new 32-bit address fields with direct access to distributed memory, data block transfer, a simple data transfer protocol and connecting of different types of existing and new devices. The new generation of 16/32-bit µP- and DSP-based systems, with high speed links, can be a new basis for development of distributed memory systems with symmetrical or non-symmetrical structures. The ways of developing such a system are presented and discussed.1. MICROCONTROLLERS, MICROPROCESSORS AND DSPsDigital control and data acquisition and processing real-time systems address a need for monitoring and control of a large number of parameters. Any simple control system includes a controller which generates actuator commands, received from an operator, and readbacks, provided by sensors. The controller processes the signals to achieve a desired response and can thus modify the frequency of response of the system. The computation element can be realized with analog or digital components. Analog controllers process a signal, can be used for very high bandwidth systems and can be realized with inexpensive components. However they suffer from component aging and temperature drift and are limited to very simple algorithms.DIGITAL CONTROLLERS approximate them, but are not affected by component aging and temperature drift and they provide stable performance with sophisticated techniques and algorithms. Programmable microcontrollers make it easy to upgrade, maintain and design systems for optimal and adaptive control. The choice of µP is critical in the performance and behavior of a digital controller. Available choices are microcontrollers, DSPs and general purpose µPs, including high cost RISC architectures. A control system requires real-time signal processing. The signal and gain coefficients must be represented accurately without loss of resolution for the smallest and largest magnitudes. Floating-point arithmetic may be necessary if gain coefficients and signals are time-varying or with large dynamic ranges [1-3].High performance is required if the sampling of signals at discrete time intervals requires high speed processing. The µP should finish the processing as soon as possible. Thus very fast instruction cycle and multiplication times are needed. Peripheral integration is important from system cost, simplicity of design and board-size points of view. Typical peripherals on such chips are I/O pins, parallel and serial interfaces, DACs and ADCs. Digital microcontrollers are primarily designed to replace hardwired logic for data acquisition and decision making in control systems. The special architecture and high performance of Digital Signal Processors (DSP) make it possible to implement a wide variety of modern Digital Controlalgorithms and Data Acquisition systems. The most popular DSP’s are from Texas Instruments, Motorola and Analog Devices.MOTOROLA has created compatible families of µPs since 1974, beginning with the 6800 family of microcontrollers, then next the 68000 line and now PowerPC RISC µPs. Motorola's DSPs, based on the DSP56000 architecture, have compatibility across an entire product line. All family members benefit from the same extended triple-bus Harvard architecture. On-Chip Emulation (OnCE) was first proposed by Motorola and is useful for field analysis and repair. Wait and stop modes reduce power consumption. The Do loop instruction directs a µP to repeat a series of operations a specified number of times without any branching time.Motorola offers 3 closely related families of DSP: DSP5610X, DSP5600X and DSP960X, along with development tools and peripherals. DSP56100 (16-bit) is optimized for the standards of digital cellular communications. The DSP56000 (24 bits) has become the standard for digital audio applications. The new member, DSP56004, is used in automobile audio systems. The DSP96000 (32 bit) family combines color graphics, sound and communications for medical, industrial and other applications. Previously limited to military, aerospace and scientific systems, DSP- technology is soon be used far more widely. TheDSP56000 family is designed as a 16-bit programmable core µP for DSPs, supplemented with configurations of memory and serial communications interfaces, timers, a host interface and in-chip coder-decoders for analog signals [1].TEXAS INSTRUMENTS produces several on-chip DSP families, including field-programmable microcontrollers and fixed- and floating-point µPs. Field Programmed Modules (FPM) are Multi-Time Programmable (MTP) µPs with EPROM and EEPROM all on a single chip. Ten year data retention,10,000 write cycles and an internal charge pump that generate a 12V programming voltage means that the end product can be adapted. The TMS370 family has more than 10 FPM members. CMOS technology gives low current consumption. The 256 bytes of EEPROM on a TMS370C710 have the advantage of being modifiable in the field. Coming from a microcontroller family these devices are well suited to industrial applications. The sensor signal µP family TMS400 was developed to serve sensor signal conditioning applications with high accuracy and precision, where average system power must be in the microwatt range [2].The FIXED POINT 16-BIT DSPs from TI give the lowest cost per MIPS in their class. TheTMS320C5X generation of DSPs gives 50 MIPS of performance at 5V and 40 MIPS at 3V. A parallel logic unit provides high speed without modifying the ALU status or registers. Software wait states provide an external interface for use of slower off-chip memory and I/O.The FLOATING POINT 32-bit DSP TMS320C30 generation integrates system control and intensive math functions for fast data movement and high-speed data processing. Powerful instructions, parallelism and buses provide flexibility in performance up to 50 MFLOPS. A high degree of parallelism allows one to perform up to 10 operations in a single instruction cycle (for example 2 data accesses, a multiply, an ALU operation and a DMA). The TMS320C32 is an enhanced low cost 32-bit DSP manufactured in 0.72 µm EPICS CMOS technology. The C32 includes all the features associated with a general purpose controller (similar to a RISC/CISC µP), but also provides additional ones. The TMS320C40 generation of DSP is effective for intensive parallel data processing in real time and has on-chip communication ports and support for shared global memory and its own pair of extended buses which give direct processor-to-processor accesses. The device provides scalability, fault tolerance and reduced bus saturation.ANALOG DEVICES INCORPORATED (ADI) has digital signal processing experience, including Mixed-Signal Peripherals (MSPeripherals) and Mixed-Signal Processors (MSProcessors). In 1982 ADI introduced the first DSP in CMOS technology with fixed- and floating-point building blocks, program sequencers, data address generators and register files. The first single chip CMOS DSP ADSP-2100 was introduced in 1986. The company defines a digitally integrated approach to analog systems of signal processing as a first step in a natural evolution in the area of Microcomputers (µC). There are two compatible ADI signal processor families: the 16-bit fixed point ADSP-2100 and 32-bit floating point ADSP-21000s. High performance serial ports (SPORTs) are bi-directional, double buffered interfaces and feature user configurable clocking, framing and word length. A Parallel Host Interface Port serves as an asynchronous interface between the signal and host µP, acting as a block of 8 dual ported registers (8/16 bit interface). An ADSP-2181 can respond to 11 interrupts (up to 6 external). Two Serial ports, SPORT0 and SPORT1, allow multiprocessor communications with word length from 3 - 16 bits (bi-directional) [3].THhe FLOATING POINT ADSP-21000 family has a complete set of arithmetic operations, including min/max, y/x etc. The parallel 9 port general purpose data register file transfers up to 9 operands to and from the computation units and memory. The program sequencer supports zero overhead looping, single cycle setup and exit for multiple program loops and delayed and nondelayed branching. The DSP-21020handles 32-bit IEEE floating point, 40-bit IEEE floating point and 32-bit fixed point data formats. System tests and on-chip emulations are provided by an IEEE JTAG boundary scan serial port. The ADSP-21010 is a low cost 32-bit version of the ADSP-21020.The SUPER HARVARD ARCHITECTURE COMPUTER ADSP-21060/62 family (SHARC) consists of 32-bit DSP microcomputers optimized for high per-formance applications, built on the same ADSP-21000 core (system on-chip), adding a dual ported on-chip SRAM and integrated I/O peripherals supported by a dedicated I/O bus. ADSP-210xx have a 25ns instruction cycle time operating at 40 MIPS. The SHARC core is compatible with the ADSP-21020.2. BUS AND NON-BUS STRUCTURESThere are bus and nonbus approaches for data acquisition and control architectures. The bus one is based on parallel µP-system interconnections for SMP; the non-bus one can be based on switches and SCI standards for Massively Parallel Processing (MPP), Supercomputers and high-speed DACs.CROSSBAR SWITCHES FOR DATA ACQUISITION can provide [1] very high-speed data processing on distributed systems and networks. There are packet switching, message passing and shared memory µP-systems for pipelined data processing and MPP. A crossbar interconnection is one of the ways for supporting multiple µPs sharing common memory having access time comparable with µP cycle times. As seen in the results of AT&T's Crossbars, these systems allow one to utilize maximum configurations and provide up to 30% improved performance over normal switching systems. The probability of successful connection of a µP to any memory unit is a function of the ratio of the number of µPs (N) and memory units (M), which function decreases to some asymptotic value as N/M. Crossbar-based systems provide, for instance, 15% better CPI value (the number of cycles per instruction) for four individual µPs having four memory units, as compared to similar bus-configured systems. Switch based systems can be constructed as either small or large networks with packet switching [4].SMALL-SCALE NETWORKS are useful in a wider range of systems than MPP, particularly applications in which I/O is dominant. Fast buses are not very cost effective because every bus slot must have all the high-speed electronics, though they may use it only a fraction of the time. In small systems many parts can be used simultaneously by different processors. Each part can be made to a narrow width and the requirements for a low-cost high-speed interface can be met by full duplex serial connections. One such approach is DS-link (from SGS-Tompson Microelectronics) for Transputers, an asynchronous serial protocol. A serial bit-stream together with its clock is coded onto 2 wires. Each DS-link requires 2 signals in each direction.A PACKET SWITCH based on STC104 is a 'wormhole' router with a 32-bit duplex DS-link interface, supporting locally adaptive routing. It consists of 32 link slices, each of which provides an input and output port. The IMS 9000 transputer integrates 4 serial link interfaces together with a superscalar µP, FPU, cache and memory interface [5]. The virtual channel processor accepts high-level communication commands from the µP and translates them into sequences of packets. A packet starts with a header and contains at most 32 bytes of data. The STS101 implements a DS-link and provides a parallel interface to other 16/32-bit µPs (synchronously or asynchronously).A LARGE NETWORK with packet switching is under research and development at CERN for future generation experiments. The IEEE P13335 standard covers the connections, cables, and electrical and logical protocols for serial interconnections, operating at speeds of 100 Mbit/s and 1 Gbit/s over copper/optical fibers. This standard has been developed in the Open Microprocessor Systems Initiative/Heterogeneous Interconnect Project (OMI/HIC). It has a goal of constructing modular, scalable, parallel low-cost systems, which support high-performance communications and transparent implementations of high level protocols. The standard is based on the STC101 and STC104 packet switches, connected to 32 DC bi-directional link ports via a 32*32 non-blocking crossbar switch [6]. The ROUTER processes up to 200 Mpackets/s with a maximum bandwidth of 300 MB/s and latency of less than 1 µs. As result they construct very large networks with different topologies (mesh, grid, tree, Clos) and investigate traffic, deliverery of packets and latency. Packets are dispatched according to some predetermined schedule. A cost-effective solution for loading, starting and monitoring the links is the T225 transputer, handling up to 4 links at a time at full bandwidth. The OS-links from that controller are used to connect nodes to the host. One implementation is being made in a study for a second level trigger. The samples of data from a detector are buffered in memory units and passed to a µP farm. A local system uses a TMS320C40 DSP for combined buffer management and local processing. This system is built around DSP-links with switching by DS-links. TheTMS320C44 DSP can receive data from up to 4 DSP-links. This special processing machine is underconstruction and has 3 stages of routing chips. The first and the third stages of switching have 112 DS-links connected to a T9000. The second stage supports 64 concurrent circuits.SCI-based modular systems can join many PCs or workstations and clusters of µPs in Multi-Processor (SCI-LAMP) Architectures. Each node in this network has a local µP, cache, memory and I/O. The local memory is distributed into private and exported portions. Private memory is used only locally, and does not participate in the SCI protocol. Conversely exported memory can be seen by other nodes as part of a single global physical address space supported by SCI.SCHEDULING of a distributed LAN is traditionally based on a centralized controller (such as a Condor OS) and message passing. Alternatively SCI can provide physically shared memory and cache-coherence among workstations across a network. Scheduling parallel jobs on a network of workstations introduces an additional level of complexity . The fast process migration and choosing of idle µPs are important to parallel performance. The number of µPs in multiprocessor systems assigned to an application might have to change when another application arrives or departs or when the degree of parallelism changes within an application. Better utilization than for fixed static partitioning is achieved with dynamic partitioning of µPs to parallel jobs. If the application cannot adjust its number of tasks during execution, several tasks from the same application may have to share a µP, introducing context switching and other related overhead. An approach to a distributed dynamic µP allocation scheme with even-loading considerations for such jobs in an SCI-LAMP system has been proposed by Li [8].3. SCI-BASED DISTRIBUTED SYSTEMSDevelopment of the first modular systems, beginning from simple register-oriented interfaces, was based on micro-computers and input-output devices for measurement, acquisition and control tasks. Bus-oriented architecture was the rule for both instrumentation and data processing. A single controller system was the rule for parallel bus (CAMAC-DW/BHW, HP-IB) and serial loop (CAMAC-SHL, HP-IL) structures. Many existing register-oriented protocols were used for measurement (HP-IB, HP-IL), data acquisition (CAMAC), control (FIELDBUS), robotics (BITBUS) and Instrumentation. Input-output subsystems [9] must be developed according to measurement, acquisition and control requirements (short address fields, direct control, data block acquisition and a simple data transfer protocol for connecting different existing and new devices). Next generation systems need a new approach to system construction based on distributed memory and modern microprocessors [10,11].The new generation distributed microprocessor systems can be divided as follows into main subsystems: a) distributed data-flow processing interconnects; b) distributed memory links; c) distributed input-output/instrumentation links. The traditional means of interconnecting is parallel bus or serial interface. But many new possibilities can be used once one has ring interconnections. The SCI-type systems are one of the best ways to construct a shared memory system. The distributed memory subsystem can be based on a RAMLINK, which is now under development. It is a single controller subsystem with a simpler protocol than SCI, which can be used as an interface for control of object-oriented single-controller systems (fieldbus/fieldring).The resulting document on SCI was approved by the IEEE on March 18, 1992. The study group concluded that any practical solution would involve the use of packet-based signaling over many independent point-to-point links, would eliminate the bus bottleneck problem and would address the problem of how to maintain cache coherence. The old concept of a single- or few-processor supercomputer has become uneconomical. The cost per computing operation is less with µP technology, so the goal of system research for some years has been to divide problems and spread them across large numbers of inexpensive distributed µPs. The simplest way is loosely coupled µPs, using many small ones each with its own memory, that pass data via "message passing".The general model will be as in a shared memory system, which may be divided into pieces and distributed as are the µPs. To reduce the effective access time to this memory cache memories keep copies of the most needed data near each µP (copies of parts of shared memory). Thus it is easy to pass data in shared memory. This model needs "cache coherent" protocols to support distributed µPs with shared memory. Among different ways of interconnecting are ringlets and switch connections. To support cost-effectively efficient coherence protocols, µPs are expected to manage cache fault handling; infrequent and complex updates would be done in software, but frequent cache updates would be done in hardware.To reduce the cost of low-end systems, SCI's nodes support a ringlet connection. This requires some extra circuitry for address recognition and buffering in each node, but makes it possible to build inexpensive SCI systems. A wide range of applications can cover the whole range from high-end µPs and their I/O systems to workstations and LANs. Very efficient LANs have been built using this model, and the usuallayers of network protocols can be added on top for compatibility with existing software. A distributed SCI system shares a 64-bit address space, where the high-order 16 bits are used to route packets to the appropriate nodes. The interconnections can be powerful switches or simple bridges that route packets between ringlets. The interconnects merely forward packets, and need know nothing about cache coherence. The initial links were 1 GB/s (16-bit 2 ns rate, differential ECL) and 1 Gbit/s (fiber optics up to a few km, coaxial cable up to about 20 m).SCI packet protocols maintain cache coherence, providing for flow control, mutual exclusion, and forward-progress guarantees needed to support multiprocessor system software and applications. Support for message-passing is an important subset of these goals. The CSR work (IEEE Std 1212-1991) was started as part of SCI. A joint approach for Futurebus+ and SCI was developed, a modular metric mechanical packaging standard IEEE Std 1301-1991, which provides physical interchangeability for SCI modules from multiple vendors. A bridge between SCI and VME (IEEE1014) will be one of the resulting commercial products.SCI is a replacement for computer buses, intended for the next generation of systems ranging from multiprocessors to workstations and PCs. But present data transfer devices are poorly matched to high performance memory systems. The low bandwidth at the I/O of the RAM packages forces one to use a wide array of RAM switch interleaving. The most effective possibility uses LVDS to connect RAMLINK chips in small rings with one controller per ring, which can interface a ring to SCI and schedules all ring activity. The controller uses a split-response packet protocol. The response time may be given either as a precise time or as an upper bound. The RAMLINK protocols simplified the SCI protocol, which can run at 500 MB/s.Interfaces between the SCI-based tightly-coupled portion of a system and other modular systems (VME, FB+, PCI), serial buses or SCI-type I/O buses are based on bridges. Local I/O devices should share µP memory address space, but peripherals may be located on separate buses. Closely coupled bridges are intended to interconnect multiple workstations and servers within a LAN. A module can consist of several linc (interface chip) interfaces (one for each bus) and could be a block in a µP. One could also be a switching component for a wireless bus.To develop a version of the RT-SCI standard optimized for real-time applications is one of the new problems of distributed systems. For RT systems latency is more important than throughput. The SCI mechanism can be replaced by a strict priority one which ensures meeting application deadlines under specified conditions. Some applications can rely on Rate Monotony Scheduling, which requires that latency be a function of priority. Thus the next levels of IEEE standards are being developed [12-14].- P1596.1: The specification defines SCI/VME bridge architectture for interfacing VME buses to an SCI. This will provide I/O support for SCI systems via VME.- P1596.2:Cache Optimizations for Large Numbers of Processors (kiloprocessors), using the SCI-developed tree-structured coherence directories and fast data distribution mechanisms for SCI systems.- P1596.3: Low-Voltage Differential Interface for SCI, specifies low-voltage differential signals for high speed communication between CMOS, GaAs and BiCMOS logic arrays, used to implement SCI. The project defined new signals that are appropriate to CMOS and other technologies to be differential with 0.25 V swing, centered on +1 V, at 2 ns signaling rate. SCI adopted the serial encoding that Hewlett-Packard devised originally for Serial HIPPI links.- P1596.4: RAMLINK (High-Bandwidth Memory Interface), based on SCI Signaling Technology, permits access to the large memory chips. This work is converging toward point-to-point links at 500MB/s using a simplified packet protocol.- P1596.5: Data Transfer Formats Optimized for SCI, defined a set of data types and formats that will work efficiently on SCI for transferring data among heterogeneous µPs in a multiprocessor SCI system.- P1596.6: SCI/RT Study Group, considers ways of getting deterministic behavior in SCI systems for real-time applications.By this time SCI has been adopted by many computer companies for internal use. Dolphin Server Technology has been formed to market SCI chips, development tools and high level models. Dolphin Interconnect Solutions' SCI-SBus adapter uses programmed I/O for messages smaller than 64 bytes. For larger messages a DMA engine conducts the data transfer . As the message size increases, a peak is reached at 85 MB/s for 64 kB messages. In its clustering of QUAD-P6 nodes, Siemens Nixdorf has announced its use of SCI as well. Among other companies which are ready to use SCI-type systems are Cray, Intel,AT&T and GIS. Cray Research's I/O channel and SCX system interconnect are moving to SCI. A newsupercomputer-class system interconnect provides high performance, scalable, reliable inter-system and system to peripheral communication (as an open standard). The SCX is ring-based and has been enhanced to address the reliability, flexibility and performance needs of distributed supercomputer environments.Much modern computing consists of a number of modular systems (PC/workstation, clusters of workstations), connected by networks. One of the best possible architectures for the nodes in SCI is that of SMP-based workstations.SUMMARYUsing the SCI standard for control systems is still expensive, and the RAMLINK protocol can be used for subsystems with distributed memory on the first level of hierarchical µP/DSP-based control systems. The complex integration of data acquisition and control can be based on the same architecture of distributed memory and direct interconnections between µP- and DSP-based nodes on a network, simplifying programming. But SCI is possible within a collaboration, using for the R&D program experimental objects of a small accelerator. The different networks for experiment data acquisition and control can be based on SCI-networks, wich connect ring- and bus-based µP subsystems.REFERENCES1. Motorola M56000, M96000 Digital Signal Processing, Motorola.2.- TMS320C50, TMS320C40 User's Guide, Texas Instruments;- MVP: The dawn of a new-era in DSP - introducing TMC320C8X,Texas Instruments.- Digital Control Applications with the TMS320; Application Notes. 1991; Texas Instruments.3. ADSP2100, ADSP21000 notes, Analog Devices Inc.4. O. Panfilov. Comparative performance analysis of crossbar switches and multiple buses.AT&T/GIS. ICSNET95 - the 11-th International Symposium. St. Petersburg. 1995.5. Peter Tompson. Small-scale Networks for General Systems Interconnect. SGS-Tompson Electronics Limited.,UK., The 11-th International Symposium ICSNET'95. St. Petersburg. 1995.6. INMOS Limited (1993). The 9000 transputer Hardware Reference Manual. SGS-Tompson Microelectronics Limited . Bristol. UK.7. R.W. Dobson, A. Martin, S. Haab, R. Heeley, M. Zhu, J. Renner Hansen. Realization of a 1000 Node High-speed Packet Switching Network. The 11-th International Symposium ICSNET'95. St. Petersburg. 1995.8. Saravanan Agasaveeran, Qiang Li. Distributed Job for Scheduling in SCI Local-Area Multiprocessor. The Fourth International Workshop on SCI-based High-performance Low-cost Computing. 1995.9. V.I. Vinogradov. Parallel-pipeline Architecture for Real-time Data-flow Acquisition in Modular Systems. IEEE Nuclear and Plasma Sciences Society. 7-th conference on computer applications in Nuclear, Particle and Plasma Physics RT'91. KFA, Germany, 1991.10.D.B. Gustavson, V.I. Vinogradov. Advanced Modular System Architecture with Distributed Memory Based on SCI. The 10-th International Symposium on problems of modular systems and networks (jubilee). ICSNET'93 Proceedings of Plenary reports, Puskin, Russia, 1993.11.D.B. Gustavson, V.I. Vinogradov. Status and Development Problems of Distributed Modular Systems Based on SCI. The 11-th International Symposium. ICSNET'95 Abstracts. St. Petersburg, 1995.12. IEEE 1596 - SCI Standard.13. IEEE P1596.4 - RAMLINK Proposal.。