Y2O3包尖晶石
尖晶石的三种结构
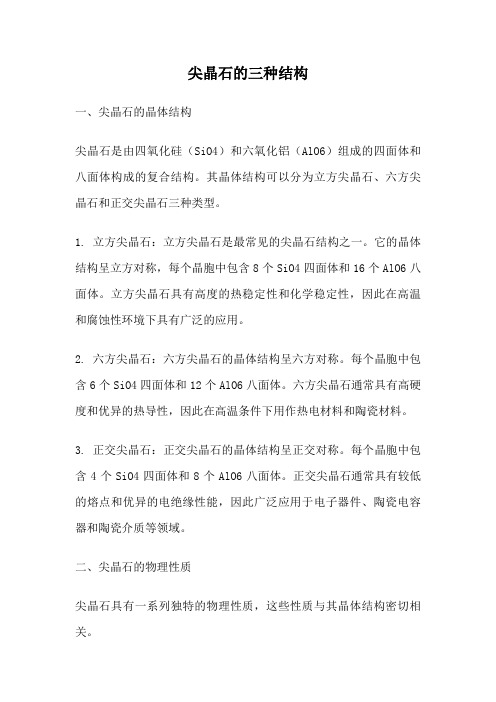
尖晶石的三种结构一、尖晶石的晶体结构尖晶石是由四氧化硅(SiO4)和六氧化铝(AlO6)组成的四面体和八面体构成的复合结构。
其晶体结构可以分为立方尖晶石、六方尖晶石和正交尖晶石三种类型。
1. 立方尖晶石:立方尖晶石是最常见的尖晶石结构之一。
它的晶体结构呈立方对称,每个晶胞中包含8个SiO4四面体和16个AlO6八面体。
立方尖晶石具有高度的热稳定性和化学稳定性,因此在高温和腐蚀性环境下具有广泛的应用。
2. 六方尖晶石:六方尖晶石的晶体结构呈六方对称。
每个晶胞中包含6个SiO4四面体和12个AlO6八面体。
六方尖晶石通常具有高硬度和优异的热导性,因此在高温条件下用作热电材料和陶瓷材料。
3. 正交尖晶石:正交尖晶石的晶体结构呈正交对称。
每个晶胞中包含4个SiO4四面体和8个AlO6八面体。
正交尖晶石通常具有较低的熔点和优异的电绝缘性能,因此广泛应用于电子器件、陶瓷电容器和陶瓷介质等领域。
二、尖晶石的物理性质尖晶石具有一系列独特的物理性质,这些性质与其晶体结构密切相关。
1. 硬度:尖晶石的硬度较高,通常在7-8级之间,因此具有良好的耐磨性和抗划伤性。
2. 密度:尖晶石的密度通常在3.4-4.1 g/cm³之间,具有适中的重量和体积。
3. 导电性:尖晶石通常是绝缘体或半导体,但某些尖晶石材料可以显示金属或半金属的导电性能。
4. 热稳定性:尖晶石具有较高的热稳定性,可以在高温环境下保持其结构和性质稳定。
5. 光学性质:尖晶石具有良好的透光性和折射性能,常被用作光学器件和宝石材料。
三、尖晶石的应用领域尖晶石由于其独特的结构和优异的物理性质,在多个领域中具有广泛的应用。
1. 电子器件:由于尖晶石具有优异的电绝缘性能和热稳定性,常被用作电子器件的基底材料、陶瓷电容器和陶瓷介质。
2. 光学器件:尖晶石具有良好的透光性和折射性能,被广泛应用于光学器件,如激光器、光纤通信和光学传感器等领域。
3. 磁性材料:某些尖晶石材料具有磁性,可用于制备磁记录材料、磁传感器和磁存储器件等。
尖晶石 结构

尖晶石结构尖晶石是一种具有特殊结构和性质的矿物,它在地质学和材料科学中有着重要的应用。
尖晶石的结构由氧离子构成的立方密堆结构和金属离子填充在其中的空隙组成,这种结构使得尖晶石具有许多独特的性质和应用。
尖晶石的化学式通常为AB2O4,其中A代表正离子,B代表二价正离子。
在尖晶石的结构中,氧离子形成了一个立方密堆结构,而A和B离子则填充在氧离子的空隙中。
这种结构使得尖晶石具有很高的结构稳定性和热稳定性,同时也赋予了它一些特殊的性质。
尖晶石具有很高的硬度和耐磨性,这使得它在工业上有广泛的应用。
例如,在陶瓷工业中,尖晶石可以用来制作高硬度和耐磨的陶瓷材料,如刀具、轴承等。
此外,尖晶石还可以用来制作电子器件中的绝缘材料和介质材料,如电容器、电感器等。
尖晶石具有很高的绝缘性能和介电常数,可以有效地隔离电子器件中的电流和信号。
除了在工业上的应用外,尖晶石还具有一些特殊的物理性质。
例如,尖晶石具有铁磁性和铁电性,这使得它在信息存储和传输方面有着重要的应用。
尖晶石可以用来制作磁性材料和铁电材料,如硬盘、磁带等。
此外,尖晶石还具有光学性质,可以用来制作光学器件和光学传感器。
尖晶石还具有一些其他的特殊性质和应用。
例如,尖晶石具有很高的抗辐射性能,可以用来制作核能设备中的结构材料和防护材料。
此外,尖晶石还可以用来制作化学传感器和生物传感器,用于检测和分析化学物质和生物分子。
总之,尖晶石是一种具有特殊结构和性质的矿物,它在地质学和材料科学中有着重要的应用。
尖晶石的结构由氧离子构成的立方密堆结构和金属离子填充在其中的空隙组成,这种结构使得尖晶石具有许多独特的性质和应用。
尖晶石在工业上可以用来制作陶瓷材料、电子器件、磁性材料等,在信息存储和传输、光学传感器等方面有着重要的应用。
此外,尖晶石还具有抗辐射性能和化学传感性能,可以用于核能设备、化学分析等领域。
尖晶石

尖晶石材料在催化氧化方面的应用简介:尖晶石是镁铝氧化物组成的矿物,因为含有镁、铁、锌、锰等等元素,它们可分为很多种。
尖晶石呈坚硬的玻璃状八面体或颗粒和块体。
它们出现在火成岩、花岗伟晶岩和变质石灰岩中。
尖晶石类催化剂在高级氧化中有着较为广泛的应用,尖晶石型催化剂在臭氧体系或者过硫酸盐体系中与臭氧或者过硫酸盐发生协同作用,提高体系的氧化性,对绝大部分有机物进行去除讲解,得到良好的效果。
关键词:尖晶石spinel催化cataly*氧化oxide正文:Mg-Fe尖晶石复合氧化物对苯乙烯选择氧化反应的催化性能,反应以H2O2为氧化剂时Mg-Fe尖晶石复合氧化物催化剂对苯乙烯选择氧化制苯甲醛反应的催化性能结果表明, 非化学计量比的Mg-Fe 尖晶石复合氧化物催化剂的活性优于纯 MgFe2O4 尖晶石相,苯甲醛产率达到 20%左右.在非化学计量比的Mg-Fe 尖晶石复合氧化物中掺入适量的Al3+后,可进一步提高催化活性, 苯甲醛最高产率达到 33.4%.催化剂表征数据揭示,非化学计量比的Mg-Fe和Mg-Fe-Al复合氧化物催化剂是由纳米尺度的铁酸盐尖晶石和α-Fe2O3微晶相构成的.除了非化学计量比尖晶石具有较多的缺陷结构外α-Fe2O3微晶相的存在也可能是造成非化学计量比催化剂活性高的原因之一。
早在 70 年代文献已报道, Mg-Fe 尖晶石复合氧化物对烷烃和烯烃的氧化脱氢反应有良好的催化效果。
后来又相继发现该类催化剂对醇脱氢和脱水、苯酚羟基化等反应有较好的催化性能。
Mg-Fe 尖晶石复合氧化物对以过氧化氢为氧化剂的苯乙烯液相氧化反应具有催化活性,并且与 TS-1 沸石不同,其主要产物不是苯乙醛和环氧化物 ,而是苯甲醛,其苯乙烯转化率还Fe-MCM-41催化剂.苯甲醛是医药、农药和香料等工业中的重要中间体,以苯乙烯为原料生产无氯苯甲醛是一种绿色工艺 ,值得深入研究 Mg-Fe 尖晶石复合氧化物的组成、结构、掺 Al3+等与催化性能。
Y2O3对激光熔覆修复航空发动机制件报废锻压模具组织与硬度的影响
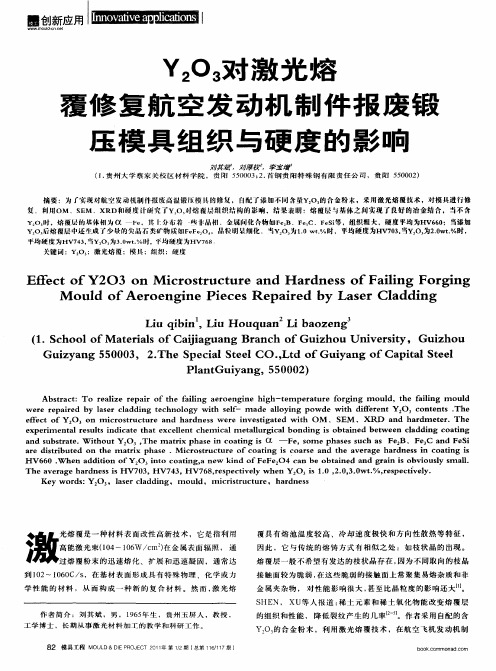
(. c o l f tr l o ia u n rn ho ih uUnv ri ,Guz o 1 S h o ei s f j g a gB a c f o Ma a Ca i Guz o ies y t ih u
慝 创新应用
ww w , oud— r net m l Gl
Y2 激 光 熔 3 o对 覆 修 复 航 空 发 动 机 制 件 报 废 锻
ห้องสมุดไป่ตู้
压 模 具 组 织 与 硬 度 的 影 响
文其斌 .吏厚权2 l j J j .李宝增 ( . 州 大 学 蔡 家 关 校 区 材 料 学 院 , 贵 阳 5 0 0 ; 首 钢 贵 阳 特 殊 钢 有 限 责 任 公 司 ,贵 阳 5 0 0 ) 1贵 5 0 3 2. 5 0 2 摘要 :为 r实现 对航空发动机制 什报 废高温锻J 模 共的修复 . 自配 了添加不 同含量Y 的合金粉 术,采用激光熔 覆技术,对模具进 行修 矗 o
e e t of Y , f c O on m i r s r c ur nd ha d s e e i ve t g t d w ih O M 、 S c o t u t e a r ne s w r n s i a e t EM 、 X RD a r nd ha dm e e .The tr e e i e a e uls i i a e t a xc le he i a e a u g c lbo i s ob a ne e w e n c a i g c tng xp rm nt lr s t nd c t h t e e l nt c m c lm t l r i a nd ng i t i d b t e l dd n oa i a u tae nd s bs r t .W ihoutY , 1。 t 0 The m a rX p s n c tng i 仅 一 Fe o e p s s s h s Fe B 、 Fe C nd Fe i t i ha e i oa i s ,s m ha e uc a 2 a S a e d s r b e n t a r x pha e .M i r t u t e o oa i s c a s nd t e a r g r e s i o tn s r i t i ut d o he m t i s c os r c ur f c tng i o r e a h ve a e ha dn s n c a i g i HV 6 0 . he d to f Y, ,i t oa i 6 W n a dii n o O n o c tng, e k nd of F Fe O 4 c n be o a ne nd gr i s o i usy s a1 a n w i e 2 a bt i d a a n i bv o l m l.
尖晶石荧光知识点总结
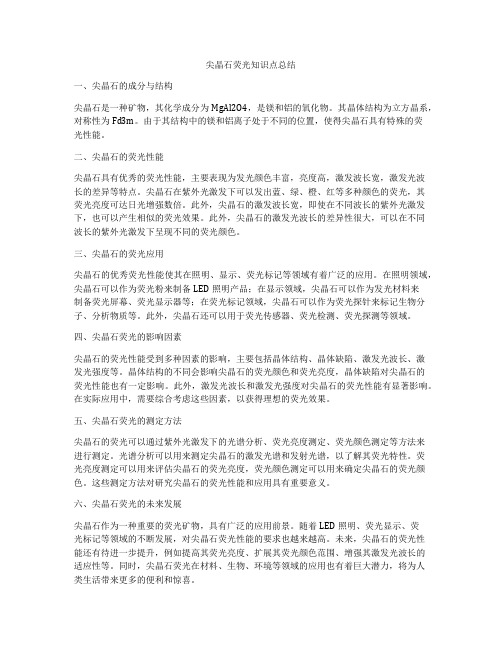
尖晶石荧光知识点总结一、尖晶石的成分与结构尖晶石是一种矿物,其化学成分为MgAl2O4,是镁和铝的氧化物。
其晶体结构为立方晶系,对称性为Fd3m。
由于其结构中的镁和铝离子处于不同的位置,使得尖晶石具有特殊的荧光性能。
二、尖晶石的荧光性能尖晶石具有优秀的荧光性能,主要表现为发光颜色丰富,亮度高,激发波长宽,激发光波长的差异等特点。
尖晶石在紫外光激发下可以发出蓝、绿、橙、红等多种颜色的荧光,其荧光亮度可达日光增强数倍。
此外,尖晶石的激发波长宽,即使在不同波长的紫外光激发下,也可以产生相似的荧光效果。
此外,尖晶石的激发光波长的差异性很大,可以在不同波长的紫外光激发下呈现不同的荧光颜色。
三、尖晶石的荧光应用尖晶石的优秀荧光性能使其在照明、显示、荧光标记等领域有着广泛的应用。
在照明领域,尖晶石可以作为荧光粉来制备LED照明产品;在显示领域,尖晶石可以作为发光材料来制备荧光屏幕、荧光显示器等;在荧光标记领域,尖晶石可以作为荧光探针来标记生物分子、分析物质等。
此外,尖晶石还可以用于荧光传感器、荧光检测、荧光探测等领域。
四、尖晶石荧光的影响因素尖晶石的荧光性能受到多种因素的影响,主要包括晶体结构、晶体缺陷、激发光波长、激发光强度等。
晶体结构的不同会影响尖晶石的荧光颜色和荧光亮度,晶体缺陷对尖晶石的荧光性能也有一定影响。
此外,激发光波长和激发光强度对尖晶石的荧光性能有显著影响。
在实际应用中,需要综合考虑这些因素,以获得理想的荧光效果。
五、尖晶石荧光的测定方法尖晶石的荧光可以通过紫外光激发下的光谱分析、荧光亮度测定、荧光颜色测定等方法来进行测定。
光谱分析可以用来测定尖晶石的激发光谱和发射光谱,以了解其荧光特性。
荧光亮度测定可以用来评估尖晶石的荧光亮度,荧光颜色测定可以用来确定尖晶石的荧光颜色。
这些测定方法对研究尖晶石的荧光性能和应用具有重要意义。
六、尖晶石荧光的未来发展尖晶石作为一种重要的荧光矿物,具有广泛的应用前景。
尖晶石知识点总结
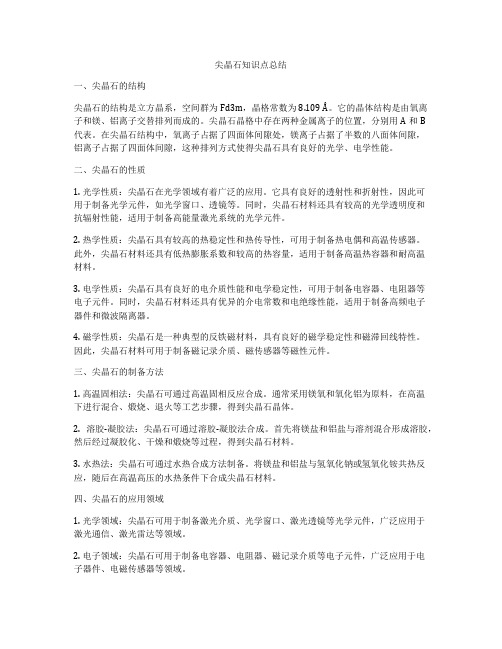
尖晶石知识点总结一、尖晶石的结构尖晶石的结构是立方晶系,空间群为Fd3m,晶格常数为8.109 Å。
它的晶体结构是由氧离子和镁、铝离子交替排列而成的。
尖晶石晶格中存在两种金属离子的位置,分别用A和B代表。
在尖晶石结构中,氧离子占据了四面体间隙处,镁离子占据了半数的八面体间隙,铝离子占据了四面体间隙,这种排列方式使得尖晶石具有良好的光学、电学性能。
二、尖晶石的性质1. 光学性质:尖晶石在光学领域有着广泛的应用。
它具有良好的透射性和折射性,因此可用于制备光学元件,如光学窗口、透镜等。
同时,尖晶石材料还具有较高的光学透明度和抗辐射性能,适用于制备高能量激光系统的光学元件。
2. 热学性质:尖晶石具有较高的热稳定性和热传导性,可用于制备热电偶和高温传感器。
此外,尖晶石材料还具有低热膨胀系数和较高的热容量,适用于制备高温热容器和耐高温材料。
3. 电学性质:尖晶石具有良好的电介质性能和电学稳定性,可用于制备电容器、电阻器等电子元件。
同时,尖晶石材料还具有优异的介电常数和电绝缘性能,适用于制备高频电子器件和微波隔离器。
4. 磁学性质:尖晶石是一种典型的反铁磁材料,具有良好的磁学稳定性和磁滞回线特性。
因此,尖晶石材料可用于制备磁记录介质、磁传感器等磁性元件。
三、尖晶石的制备方法1. 高温固相法:尖晶石可通过高温固相反应合成。
通常采用镁氧和氧化铝为原料,在高温下进行混合、煅烧、退火等工艺步骤,得到尖晶石晶体。
2. 溶胶-凝胶法:尖晶石可通过溶胶-凝胶法合成。
首先将镁盐和铝盐与溶剂混合形成溶胶,然后经过凝胶化、干燥和煅烧等过程,得到尖晶石材料。
3. 水热法:尖晶石可通过水热合成方法制备。
将镁盐和铝盐与氢氧化钠或氢氧化铵共热反应,随后在高温高压的水热条件下合成尖晶石材料。
四、尖晶石的应用领域1. 光学领域:尖晶石可用于制备激光介质、光学窗口、激光透镜等光学元件,广泛应用于激光通信、激光雷达等领域。
2. 电子领域:尖晶石可用于制备电容器、电阻器、磁记录介质等电子元件,广泛应用于电子器件、电磁传感器等领域。
尖晶石的概念
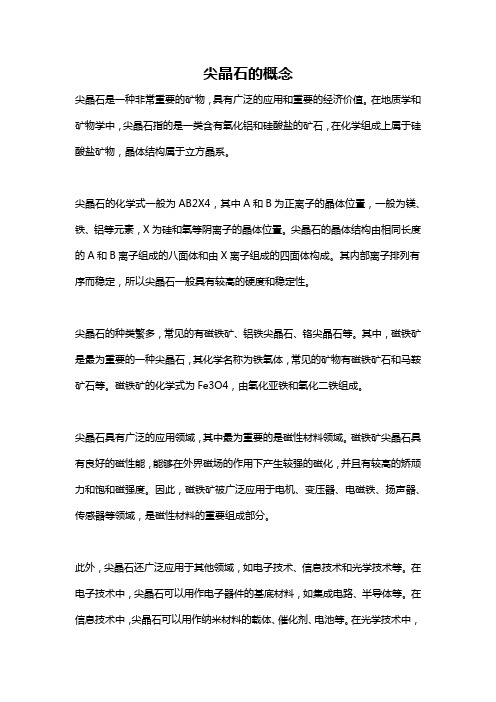
尖晶石的概念尖晶石是一种非常重要的矿物,具有广泛的应用和重要的经济价值。
在地质学和矿物学中,尖晶石指的是一类含有氧化铝和硅酸盐的矿石,在化学组成上属于硅酸盐矿物,晶体结构属于立方晶系。
尖晶石的化学式一般为AB2X4,其中A和B为正离子的晶体位置,一般为镁、铁、铝等元素,X为硅和氧等阴离子的晶体位置。
尖晶石的晶体结构由相同长度的A和B离子组成的八面体和由X离子组成的四面体构成。
其内部离子排列有序而稳定,所以尖晶石一般具有较高的硬度和稳定性。
尖晶石的种类繁多,常见的有磁铁矿、铝铁尖晶石、铬尖晶石等。
其中,磁铁矿是最为重要的一种尖晶石,其化学名称为铁氧体,常见的矿物有磁铁矿石和马鞍矿石等。
磁铁矿的化学式为Fe3O4,由氧化亚铁和氧化二铁组成。
尖晶石具有广泛的应用领域,其中最为重要的是磁性材料领域。
磁铁矿尖晶石具有良好的磁性能,能够在外界磁场的作用下产生较强的磁化,并且有较高的矫顽力和饱和磁强度。
因此,磁铁矿被广泛应用于电机、变压器、电磁铁、扬声器、传感器等领域,是磁性材料的重要组成部分。
此外,尖晶石还广泛应用于其他领域,如电子技术、信息技术和光学技术等。
在电子技术中,尖晶石可以用作电子器件的基底材料,如集成电路、半导体等。
在信息技术中,尖晶石可以用作纳米材料的载体、催化剂、电池等。
在光学技术中,尖晶石可以用作激光器和光纤放大器的工作介质。
尖晶石还具有一定的饰品和装饰用途。
例如,尖晶石的艳丽颜色和晶体结构可以用于制作首饰,如项链、手链、戒指等。
此外,尖晶石的晶体结构也可以用于制作人造宝石和天然宝石的外观增光。
尽管尖晶石在各个领域都具有广泛的应用,但是由于尖晶石的产量有限,其价格也相对较高,因此在一些应用上被更为廉价的替代材料所替代。
此外,尖晶石的开采和加工也给环境造成一定的压力,特别是在采矿过程中产生的尾矿和工业废水处理等问题。
总的来说,尖晶石是一种非常重要的矿物,具有广泛的应用和重要的经济价值。
其在磁性材料、电子技术、信息技术、光学技术和装饰等领域发挥着重要的作用。
添加纳米Y2O3或纳米ZnO对熔融石英陶瓷脱玻性能的影响
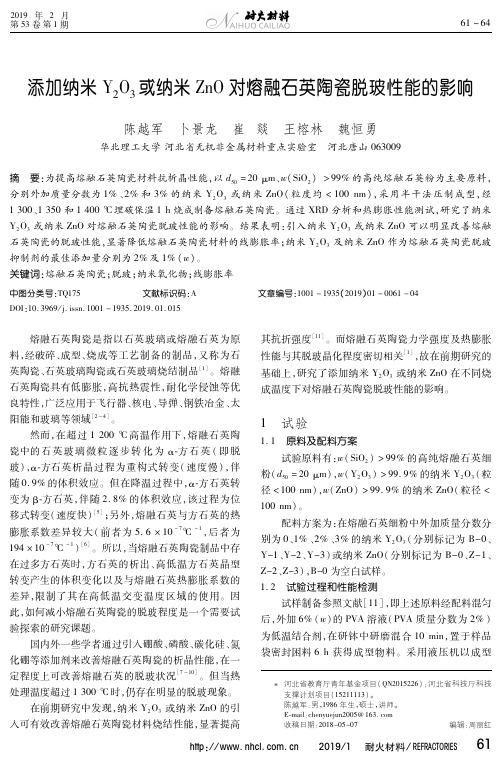
61~64
添加纳米 Y2O3或纳米 ZnO对熔融石英陶瓷脱玻性能的影响
陈越军 卜景龙 崔 邁 王榕林 魏恒勇
华北理工大学 河北省无机非要:为提高熔融石英陶瓷材料抗析晶性能,以 d50=20μm、w(SiO2) >99%的高纯熔融石英粉为主要原料, 分别外加质量分数为 1%、2% 和 3% 的纳米 Y2O3 或纳米 ZnO(粒度均 <100nm),采用半干法压制成型,经 1300、1350和 1400℃埋碳保温 1h烧成制备熔融石英陶瓷。通过 XRD分析和热膨胀性能测试,研究了纳米 Y2O3或纳米 ZnO对熔融石英陶瓷脱玻性能的影响。结果表明:引入纳米 Y2O3或纳米 ZnO可以明显改善熔融 石英陶瓷的脱玻性能,显著降低熔融石英陶瓷材料的线膨胀率;纳米 Y2O3 及纳米 ZnO作为熔融石英陶瓷脱玻 抑制剂的最佳添加量分别为 2%及 1%(w)。 关键词:熔融石英陶瓷;脱玻;纳米氧化物;线膨胀率
压力 50MPa(保压 15s)压 制 成 50mm ×10mm × 10mm坯体,坯体于 110℃干燥 24h以上,最后置于 埋有碳粉的匣钵中,分别经 1300、1350和 1400℃保 温 1h烧成。然后采用 X射线衍射仪分析烧后试样 的物相组成,采用卧式热膨胀仪按 GB/T7320—2008 测定烧后试样的线膨胀率(室温至 1000℃)。
2 结果与分析
2.1 XRD分析 1300、1350和 1400℃ 保 温 1h烧 后 试 样 的
XRD图谱分别示于图 1—图 3。
中的熔融石英已部分脱玻,脱玻晶化产物为方石英。 与试样 B-0相比,在衍射角 21.9°附近,含纳米 Y2O3 试样无方石英衍射峰,仅有微微隆起的漫散射峰(馒 头峰);试样 Z-1和试样 Z-3存在微弱的方石英衍射 峰,即试样中的物相也基本为玻璃态的熔融石英,表 明在 1300℃,纳米 Y2O3 或纳米 ZnO对熔融石英具 有明显的抑制脱玻作用。
CeO2和Sm2O3对方镁石尖晶石材料烧结性能及显微结构的影响

2.1 材料的烧结性能 稀土氧化物 CeO2和 Sm2O3添加量及烧成温度对
试样体积密度、显气孔率与线收缩率的影响见图 1。 由图 1可知:稀土氧化物 CeO2 和 Sm2O3 的添加均能 显著改善材料烧结性能;而且,添加 CeO2 的比添加 Sm2O3 的促进试样烧结致密化 的 效 果 明 显,且 随 着 添 加量的增 加,试 样 体 积 密 度 与 线 收 缩 率 都 有 明 显 升 高。由图还可以看出:1750℃时,未添加稀土氧化物 试样的 体 积 密 度 仅 为 3.03g· cm-3,显 气 孔 率 为 15.9%,烧 后 线 收 缩 率6.58% ;添 加3%(w)CeO2试
原料 尖晶石细粉
w/% SiO2 Al2O3 Fe2O3 CaO MgO Na2O 0.10 65.68 0.26 0.57 32.31 0.03
镁砂细粉
1.07 0.35 0.78 1.14 96.06
1.2 性能检测 依据 GB/T3997.1—1998测定试样烧成前后的
直径 变 化,计 算 烧 后 试 样 的 线 变 化 率。依 据 GB/T 2997—2000测量 烧 后 试 样 的 体 积 密 度 与 显 气 孔 率。 采用 X射线衍射仪测定 1750℃烧后试样的相组成。 采用扫描电子显微镜及能谱仪对 1750℃烧后试样 进行显微结构观察和微区成分分析。
中图分类号:TQ175.4 文献标识码:A 文章编号:1001-1935(2019)02-0109-04 DOI:10.3969/j.issn.1001-1935.2019.02.007
方镁石-尖晶石质耐火材料,因其高温强度大,荷 重软化温度高,抗热震性好,抗碱性渣性能优良[1-6], 常被应用在水泥窑、有色冶金等领域。然而,方镁石 与尖晶石难烧结,导致其致密化程度较低。为了提高 方镁石-尖晶石质耐火材料的烧结致密性和优化显微 结构,人们进行了诸多研究。曹林洪等[7]研究发现, 方镁石-尖晶石基质中加 TiO2会大幅度提高方镁石- 尖晶石的烧结性能,而且随着 TiO2加入量的增加,方 镁石-尖晶石的体积密度也有所增加;方镁石-尖晶石 中加入 Al2O3则能提高其耐压强度和体积密度;在方 镁石-尖晶石中加入 Fe2O3能提高常温耐压强度和改 善烧结性能。
尖晶石材料的新功能与应用
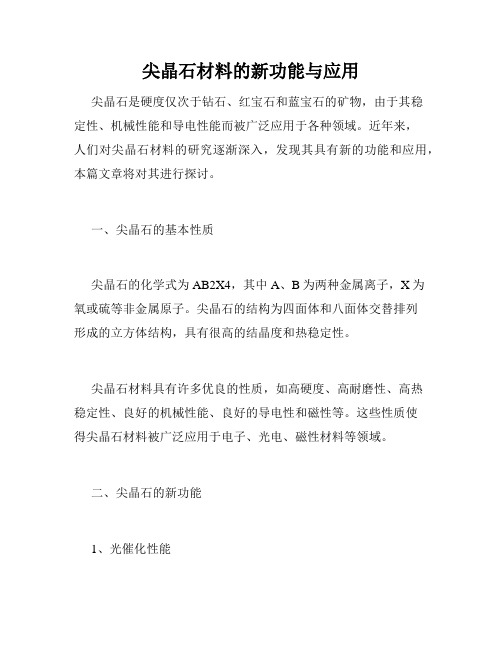
尖晶石材料的新功能与应用尖晶石是硬度仅次于钻石、红宝石和蓝宝石的矿物,由于其稳定性、机械性能和导电性能而被广泛应用于各种领域。
近年来,人们对尖晶石材料的研究逐渐深入,发现其具有新的功能和应用,本篇文章将对其进行探讨。
一、尖晶石的基本性质尖晶石的化学式为AB2X4,其中A、B为两种金属离子,X为氧或硫等非金属原子。
尖晶石的结构为四面体和八面体交替排列形成的立方体结构,具有很高的结晶度和热稳定性。
尖晶石材料具有许多优良的性质,如高硬度、高耐磨性、高热稳定性、良好的机械性能、良好的导电性和磁性等。
这些性质使得尖晶石材料被广泛应用于电子、光电、磁性材料等领域。
二、尖晶石的新功能1、光催化性能近年来,尖晶石材料在光催化领域获得了广泛关注。
由于其化学稳定性和高的光催化性能,在环境污染物降解、水分解制氢等领域具有很大的潜力。
研究发现,尖晶石材料的光催化活性主要受晶面结构、晶格偏压和表面缺陷等因素的影响。
2、电化学性能尖晶石材料在电化学领域也有广泛应用,具有良好的电化学稳定性和高的电导率。
研究人员通过改变材料的结构和组分,设计了多种尖晶石电极材料,用于超级电容器、电容器、锂离子电池等领域。
3、光学性能由于尖晶石晶体的特殊结构和高的透光性,可以用于制备各种光学元件。
研究表明,尖晶石材料在可见光和红外光区域具有较大的折射率和色散率,可以用于制备透镜、光学波导等。
三、尖晶石的应用1、高温结构材料尖晶石由于其高的热稳定性和机械强度,加上良好的防氧化性能,可以用于高温结构材料的制备。
例如,尖晶石陶瓷材料可以制备用于高温燃气轮机和航空发动机的涡轮叶片。
2、磁性材料尖晶石中的B离子可以形成具有磁性的晶格结构,因此可以用于制备各种磁性材料。
研究人员还发现,通过改变尖晶石材料的组分和结构,可以制备出各种形状和大小的磁性纳米晶体,这些纳米晶体在储存和传输信息等领域具有广泛应用。
3、光电材料尖晶石具有良好的光学和电学性质,可以用于制备各种光电材料。
《含纳米Y2O3过共晶Fe-Cr-C合金显微组织与耐磨性研究》
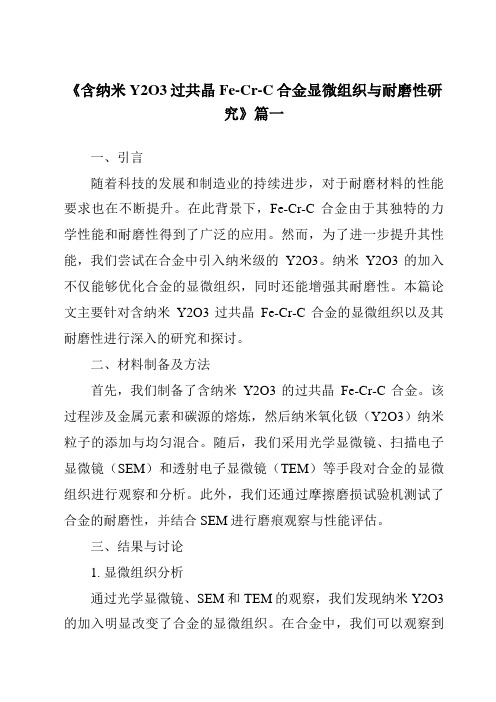
《含纳米Y2O3过共晶Fe-Cr-C合金显微组织与耐磨性研究》篇一一、引言随着科技的发展和制造业的持续进步,对于耐磨材料的性能要求也在不断提升。
在此背景下,Fe-Cr-C合金由于其独特的力学性能和耐磨性得到了广泛的应用。
然而,为了进一步提升其性能,我们尝试在合金中引入纳米级的Y2O3。
纳米Y2O3的加入不仅能够优化合金的显微组织,同时还能增强其耐磨性。
本篇论文主要针对含纳米Y2O3过共晶Fe-Cr-C合金的显微组织以及其耐磨性进行深入的研究和探讨。
二、材料制备及方法首先,我们制备了含纳米Y2O3的过共晶Fe-Cr-C合金。
该过程涉及金属元素和碳源的熔炼,然后纳米氧化钑(Y2O3)纳米粒子的添加与均匀混合。
随后,我们采用光学显微镜、扫描电子显微镜(SEM)和透射电子显微镜(TEM)等手段对合金的显微组织进行观察和分析。
此外,我们还通过摩擦磨损试验机测试了合金的耐磨性,并结合SEM进行磨痕观察与性能评估。
三、结果与讨论1. 显微组织分析通过光学显微镜、SEM和TEM的观察,我们发现纳米Y2O3的加入明显改变了合金的显微组织。
在合金中,我们可以观察到大量的硬质相颗粒,这些颗粒被均匀地分散在基体中。
硬质相的存在使合金在摩擦过程中具有更好的承载能力,有助于提高其耐磨性。
此外,纳米Y2O3的加入还使得合金的晶粒更加细化,这也有助于提高其整体力学性能。
2. 耐磨性研究通过摩擦磨损试验机对含纳米Y2O3过共晶Fe-Cr-C合金进行测试,我们发现其耐磨性得到了显著提高。
在相同条件下,与未添加纳米Y2O3的合金相比,该合金的磨损率明显降低。
这主要归因于硬质相颗粒的分散分布以及纳米Y2O3对晶粒细化的作用。
硬质相颗粒能够有效地承受摩擦力,降低磨损;而晶粒细化则能够提高合金的整体强度和韧性,使其在摩擦过程中不易发生断裂和脱落。
四、结论本研究通过引入纳米Y2O3,成功优化了过共晶Fe-Cr-C合金的显微组织,显著提高了其耐磨性。
《2024年含纳米Y2O3过共晶Fe-Cr-C合金显微组织与耐磨性研究》范文
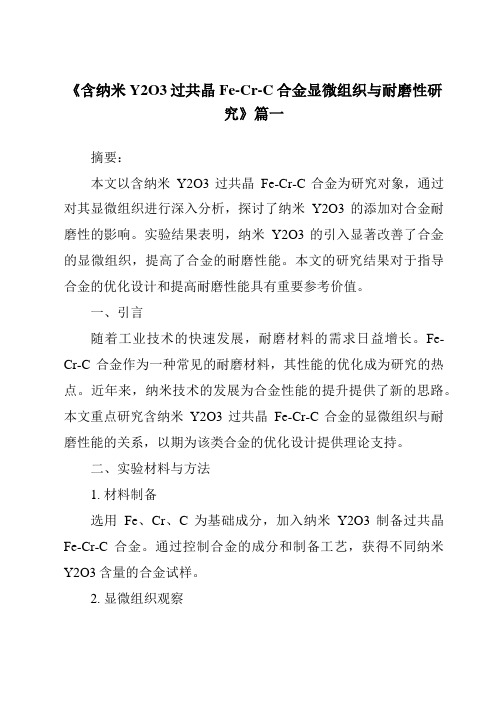
《含纳米Y2O3过共晶Fe-Cr-C合金显微组织与耐磨性研究》篇一摘要:本文以含纳米Y2O3过共晶Fe-Cr-C合金为研究对象,通过对其显微组织进行深入分析,探讨了纳米Y2O3的添加对合金耐磨性的影响。
实验结果表明,纳米Y2O3的引入显著改善了合金的显微组织,提高了合金的耐磨性能。
本文的研究结果对于指导合金的优化设计和提高耐磨性能具有重要参考价值。
一、引言随着工业技术的快速发展,耐磨材料的需求日益增长。
Fe-Cr-C合金作为一种常见的耐磨材料,其性能的优化成为研究的热点。
近年来,纳米技术的发展为合金性能的提升提供了新的思路。
本文重点研究含纳米Y2O3过共晶Fe-Cr-C合金的显微组织与耐磨性能的关系,以期为该类合金的优化设计提供理论支持。
二、实验材料与方法1. 材料制备选用Fe、Cr、C为基础成分,加入纳米Y2O3制备过共晶Fe-Cr-C合金。
通过控制合金的成分和制备工艺,获得不同纳米Y2O3含量的合金试样。
2. 显微组织观察利用金相显微镜、扫描电子显微镜(SEM)和透射电子显微镜(TEM)对合金的显微组织进行观察,分析纳米Y2O3在合金中的分布和形态。
3. 耐磨性测试采用磨损试验机对合金试样的耐磨性能进行测试,比较不同纳米Y2O3含量合金的耐磨性能。
三、结果与讨论1. 显微组织分析(1)金相显微镜观察结果显示,纳米Y2O3的加入使合金的组织更加致密,晶粒尺寸减小。
(2)SEM和TEM观察发现,纳米Y2O3在合金中呈现均匀分布,与基体形成了良好的界面结合。
2. 耐磨性分析(1)随着纳米Y2O3含量的增加,合金的耐磨性能呈现先增加后稳定的趋势。
适量纳米Y2O3的加入能够有效提高合金的硬度,从而增强其耐磨性能。
(2)纳米Y2O3的加入改善了合金的摩擦学性能,减少了磨损过程中的摩擦系数,有效抑制了磨损过程的进行。
四、结论本文研究表明,纳米Y2O3的引入能够显著改善Fe-Cr-C合金的显微组织,使其组织更加致密,晶粒尺寸减小。
《含纳米Y2O3过共晶Fe-Cr-C合金显微组织与耐磨性研究》范文
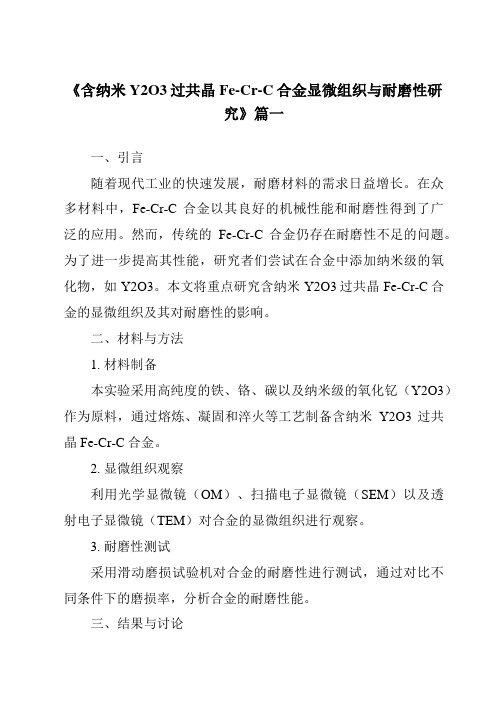
《含纳米Y2O3过共晶Fe-Cr-C合金显微组织与耐磨性研究》篇一一、引言随着现代工业的快速发展,耐磨材料的需求日益增长。
在众多材料中,Fe-Cr-C合金以其良好的机械性能和耐磨性得到了广泛的应用。
然而,传统的Fe-Cr-C合金仍存在耐磨性不足的问题。
为了进一步提高其性能,研究者们尝试在合金中添加纳米级的氧化物,如Y2O3。
本文将重点研究含纳米Y2O3过共晶Fe-Cr-C合金的显微组织及其对耐磨性的影响。
二、材料与方法1. 材料制备本实验采用高纯度的铁、铬、碳以及纳米级的氧化钇(Y2O3)作为原料,通过熔炼、凝固和淬火等工艺制备含纳米Y2O3过共晶Fe-Cr-C合金。
2. 显微组织观察利用光学显微镜(OM)、扫描电子显微镜(SEM)以及透射电子显微镜(TEM)对合金的显微组织进行观察。
3. 耐磨性测试采用滑动磨损试验机对合金的耐磨性进行测试,通过对比不同条件下的磨损率,分析合金的耐磨性能。
三、结果与讨论1. 显微组织分析通过OM、SEM和TEM观察发现,含纳米Y2O3的过共晶Fe-Cr-C合金具有明显的显微组织特征。
纳米Y2O3的加入使得合金中出现了更多的硬质相,这些硬质相有效地提高了合金的硬度。
此外,纳米Y2O3还起到了细化晶粒的作用,使得合金的显微组织更加均匀。
2. 耐磨性分析实验结果表明,含纳米Y2O3的过共晶Fe-Cr-C合金具有较高的耐磨性。
与未添加纳米Y2O3的合金相比,其磨损率明显降低。
这主要归因于纳米Y2O3的加入使得合金中硬质相增多,提高了合金的硬度,从而增强了其抵抗磨损的能力。
此外,细化的晶粒也有利于提高合金的耐磨性。
四、结论本研究表明,含纳米Y2O3的过共晶Fe-Cr-C合金具有优异的显微组织和耐磨性能。
纳米Y2O3的加入使得合金中硬质相增多,晶粒细化,从而提高了合金的硬度和耐磨性。
因此,含纳米Y2O3的过共晶Fe-Cr-C合金在工业领域具有广泛的应用前景。
五、展望未来研究可进一步探讨纳米Y2O3的添加量对Fe-Cr-C合金显微组织和耐磨性的影响,以及在不同工况下合金的耐磨性能。
《2024年含纳米Y2O3过共晶Fe-Cr-C合金显微组织与耐磨性研究》范文
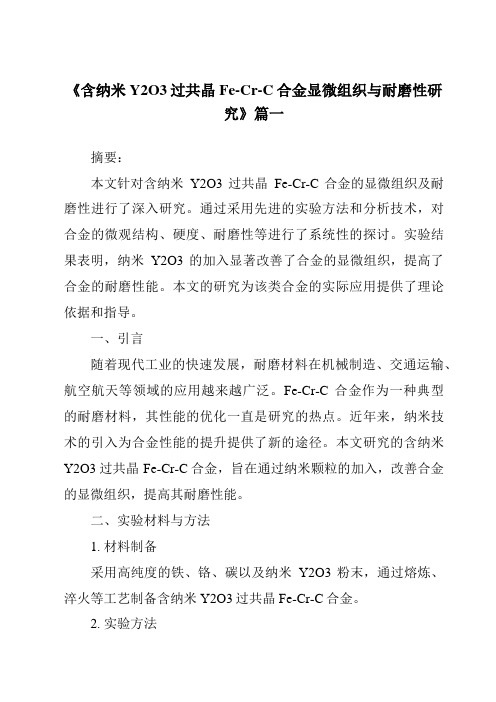
《含纳米Y2O3过共晶Fe-Cr-C合金显微组织与耐磨性研究》篇一摘要:本文针对含纳米Y2O3过共晶Fe-Cr-C合金的显微组织及耐磨性进行了深入研究。
通过采用先进的实验方法和分析技术,对合金的微观结构、硬度、耐磨性等进行了系统性的探讨。
实验结果表明,纳米Y2O3的加入显著改善了合金的显微组织,提高了合金的耐磨性能。
本文的研究为该类合金的实际应用提供了理论依据和指导。
一、引言随着现代工业的快速发展,耐磨材料在机械制造、交通运输、航空航天等领域的应用越来越广泛。
Fe-Cr-C合金作为一种典型的耐磨材料,其性能的优化一直是研究的热点。
近年来,纳米技术的引入为合金性能的提升提供了新的途径。
本文研究的含纳米Y2O3过共晶Fe-Cr-C合金,旨在通过纳米颗粒的加入,改善合金的显微组织,提高其耐磨性能。
二、实验材料与方法1. 材料制备采用高纯度的铁、铬、碳以及纳米Y2O3粉末,通过熔炼、淬火等工艺制备含纳米Y2O3过共晶Fe-Cr-C合金。
2. 实验方法(1)采用金相显微镜观察合金的显微组织;(2)利用X射线衍射仪分析合金的物相组成;(3)采用显微硬度计测试合金的硬度;(4)通过磨损试验机评价合金的耐磨性能。
三、结果与讨论1. 显微组织分析金相显微镜观察结果表明,含纳米Y2O3的合金显微组织得到显著改善,晶粒细化,且分布均匀。
纳米Y2O3的加入有效地阻止了晶粒的长大,提高了合金的致密度。
2. 物相分析X射线衍射分析显示,合金中主要物相为Fe、Cr的固溶体以及碳化物等。
纳米Y2O3的加入对物相组成无明显影响,但可促进碳化物的均匀分布和细化。
3. 硬度与耐磨性分析(1)硬度:与未添加纳米Y2O3的合金相比,含纳米Y2O3的合金硬度显著提高,这主要归因于晶粒细化以及碳化物的均匀分布。
(2)耐磨性:磨损试验结果表明,含纳米Y2O3的合金耐磨性能明显优于未添加纳米Y2O3的合金。
这主要得益于合金硬度的提高以及纳米Y2O3对磨损过程中产生的微裂纹的阻碍作用。
《含纳米Y2O3过共晶Fe-Cr-C合金显微组织与耐磨性研究》范文
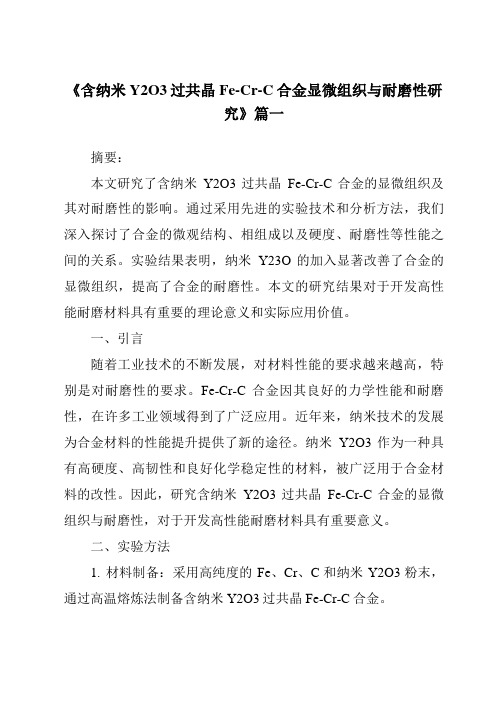
《含纳米Y2O3过共晶Fe-Cr-C合金显微组织与耐磨性研究》篇一摘要:本文研究了含纳米Y2O3过共晶Fe-Cr-C合金的显微组织及其对耐磨性的影响。
通过采用先进的实验技术和分析方法,我们深入探讨了合金的微观结构、相组成以及硬度、耐磨性等性能之间的关系。
实验结果表明,纳米Y23O的加入显著改善了合金的显微组织,提高了合金的耐磨性。
本文的研究结果对于开发高性能耐磨材料具有重要的理论意义和实际应用价值。
一、引言随着工业技术的不断发展,对材料性能的要求越来越高,特别是对耐磨性的要求。
Fe-Cr-C合金因其良好的力学性能和耐磨性,在许多工业领域得到了广泛应用。
近年来,纳米技术的发展为合金材料的性能提升提供了新的途径。
纳米Y2O3作为一种具有高硬度、高韧性和良好化学稳定性的材料,被广泛用于合金材料的改性。
因此,研究含纳米Y2O3过共晶Fe-Cr-C合金的显微组织与耐磨性,对于开发高性能耐磨材料具有重要意义。
二、实验方法1. 材料制备:采用高纯度的Fe、Cr、C和纳米Y2O3粉末,通过高温熔炼法制备含纳米Y2O3过共晶Fe-Cr-C合金。
2. 显微组织观察:利用光学显微镜(OM)、扫描电子显微镜(SEM)和透射电子显微镜(TEM)对合金的显微组织进行观察。
3. 性能测试:采用维氏硬度计测试合金的硬度,通过摩擦磨损试验机测试合金的耐磨性。
三、结果与讨论1. 显微组织分析通过OM、SEM和TEM观察,我们发现含纳米Y2O3的过共晶Fe-Cr-C合金具有明显的显微组织特征。
合金中存在大量的硬质相,这些硬质相在基体中分布均匀,且与基体结合紧密。
纳米Y2O3的加入使得硬质相的数量增加,且尺寸更加细小均匀。
此外,合金的晶界清晰,没有明显的缺陷和杂质。
2. 硬度与耐磨性分析实验结果表明,含纳米Y2O3的过共晶Fe-Cr-C合金具有较高的硬度。
这是由于纳米Y2O3的加入使得硬质相的数量和尺寸增加,从而提高了合金的整体硬度。
《2024年含纳米Y2O3过共晶Fe-Cr-C合金显微组织与耐磨性研究》范文

《含纳米Y2O3过共晶Fe-Cr-C合金显微组织与耐磨性研究》篇一一、引言在当今机械工程和材料科学领域中,合金材料的显微组织及其对耐磨性的影响已成为重要研究课题。
尤其是含有稀土氧化物纳米粒子的过共晶合金,由于其独特性质和潜在的实用价值,已成为科研工作者研究的热点。
本研究旨在深入探讨含纳米Y2O3的过共晶Fe-Cr-C合金的显微组织及其对耐磨性的影响。
二、材料与方法1. 材料制备本实验采用高纯度的铁、铬、碳元素为基础,添加纳米级Y2O3粉末,通过熔炼法制备过共晶Fe-Cr-C合金。
2. 显微组织观察利用光学显微镜(OM)、扫描电子显微镜(SEM)和透射电子显微镜(TEM)对合金的显微组织进行观察。
3. 耐磨性测试采用磨损试验机对合金的耐磨性进行测试,通过比较磨损率及磨损表面的形貌变化来评价其耐磨性能。
三、结果与讨论1. 显微组织分析实验观察到含纳米Y2O3的过共晶Fe-Cr-C合金中,Y2O3纳米粒子均匀地分布在基体中,形成了一种特殊的复合结构。
这种结构中,硬质相与软质相的分布更加均匀,有效提高了合金的力学性能。
2. 耐磨性分析通过耐磨性测试发现,含纳米Y2O3的过共晶Fe-Cr-C合金相较于未添加Y2O3的合金表现出更高的耐磨性。
其磨损率显著降低,且磨损表面形貌更为平滑,没有明显的磨屑堆积和裂纹扩展。
这表明纳米Y2O3的添加有效提高了合金的耐磨性能。
四、显微组织对耐磨性的影响机制探讨1. 硬质相与软质相的协同作用在含纳米Y2O3的过共晶Fe-Cr-C合金中,硬质相(如Fe-Cr 复合物)和软质相(如基体)之间的协同作用得到了有效发挥。
硬质相能够承受较大的压力和摩擦力,而软质相则能提供良好的韧性和延展性,二者共同作用使得合金具有更好的耐磨性。
2. 纳米Y2O3的作用纳米Y2O3的添加使得合金中形成了大量的微小颗粒,这些颗粒在摩擦过程中能够起到润滑作用,减少摩擦系数和磨损率。
此外,纳米Y2O3还能有效提高合金的硬度,进一步增强其耐磨性能。
《含纳米Y2O3过共晶Fe-Cr-C合金显微组织与耐磨性研究》
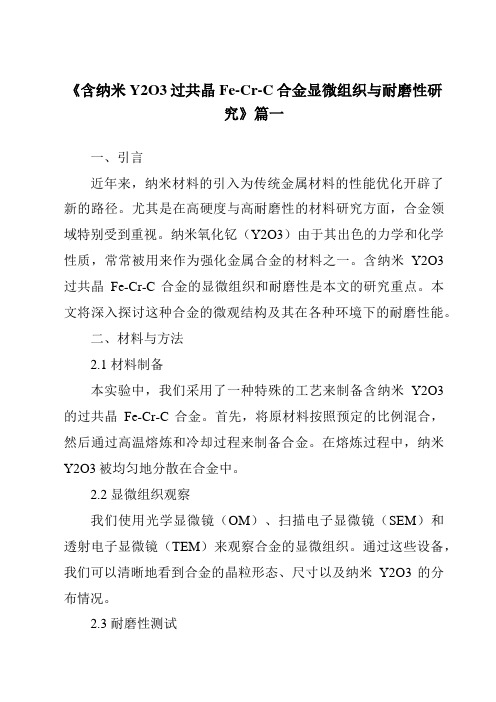
《含纳米Y2O3过共晶Fe-Cr-C合金显微组织与耐磨性研究》篇一一、引言近年来,纳米材料的引入为传统金属材料的性能优化开辟了新的路径。
尤其是在高硬度与高耐磨性的材料研究方面,合金领域特别受到重视。
纳米氧化钇(Y2O3)由于其出色的力学和化学性质,常常被用来作为强化金属合金的材料之一。
含纳米Y2O3过共晶Fe-Cr-C合金的显微组织和耐磨性是本文的研究重点。
本文将深入探讨这种合金的微观结构及其在各种环境下的耐磨性能。
二、材料与方法2.1 材料制备本实验中,我们采用了一种特殊的工艺来制备含纳米Y2O3的过共晶Fe-Cr-C合金。
首先,将原材料按照预定的比例混合,然后通过高温熔炼和冷却过程来制备合金。
在熔炼过程中,纳米Y2O3被均匀地分散在合金中。
2.2 显微组织观察我们使用光学显微镜(OM)、扫描电子显微镜(SEM)和透射电子显微镜(TEM)来观察合金的显微组织。
通过这些设备,我们可以清晰地看到合金的晶粒形态、尺寸以及纳米Y2O3的分布情况。
2.3 耐磨性测试耐磨性测试是通过在特定的磨损试验机上进行。
我们采用了多种磨损条件,包括干摩擦、水润滑和油润滑等,以全面评估合金的耐磨性能。
三、结果与讨论3.1 显微组织分析通过OM、SEM和TEM的观察,我们发现含纳米Y2O3的过共晶Fe-Cr-C合金具有均匀的晶粒结构和良好的组织致密度。
纳米Y2O3在合金中分布均匀,有效地强化了合金的基体。
此外,我们还观察到了一些硬质相的形成,这些硬质相的存在进一步提高了合金的硬度。
3.2 耐磨性分析在干摩擦条件下,含纳米Y2O3的过共晶Fe-Cr-C合金表现出优异的耐磨性。
这主要归因于其高硬度和良好的组织致密度。
在水润滑和油润滑条件下,这种合金的耐磨性也有显著的提高。
这可能是由于润滑剂有效地减少了摩擦表面的摩擦力,从而降低了磨损率。
此外,纳米Y2O3的存在也起到了增强润滑剂附着力和提高润滑效果的作用。
四、结论本文研究了含纳米Y2O3过共晶Fe-Cr-C合金的显微组织和耐磨性。
Y2O3对氧化锆陶瓷组织和性能的影响
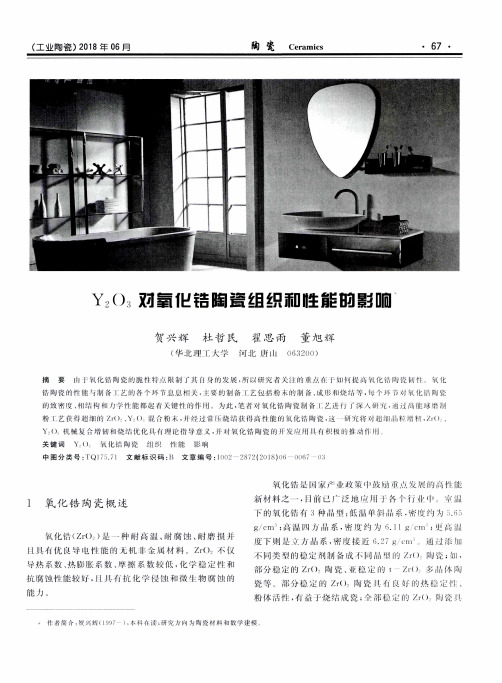
小 同 类 J 的 稳 定 齐IJ 衙 成 1 I ¨洲 Zr() 瓷 ; .
部 分 稳 定 的 Z ‘() 陶 瓷 、、 正的 I /r() 彩 f f4 陶
瓷 等 。 部 分稳 定 的 Zr() 陶 瓷 !I
的 热 trf 、
粉 休 活 r .彳J‘i盎J 烧 。‘ JJ 瓷 ;个: f5 ff0 Zr(J J j l l
新 材 料 之 一 .『I lji『 』 泛 地 川 j
、『 Il』 I
下 的 氧 化竹 仃 {种 f}fI J :低 .{ p斜 j 乐 .惭 约 .“
/CII3_ ;I 濉 【Ju,J‘}1『l系 .擀 J耍约 【I ¨·l; f ’ m
发 下 则 址 ● ‘【1^系 . 艘 接 近 【;.17 g 11 迎 过 涂 JJIJ
(工业陶瓷)2018年06月 源自陶 瓷 Ceramics
· 67 ·
Y2() 对 萤 化 锆 陶 瓷 组 织 和 n生鹾 的 影 响
贺 兴辉 杜 哲 民 翟 思 雨 董 旭 辉
(华 北 人 学 河 』七 唐 1I J 0632()(1)
摘 要 Itl j‘钣 化 竹 陶 瓷的 憷 特 点 制 』 『J身 的 发 ,所 以研 究 告 父 的 币,· r ¨ 捉 『 代 化 陶 瓷 rI 讯 化
关 键 词 Y ()、 议 化 陶 瓷 i ! r{:能 影 响
中 图分 类 号 :TQ1 . 1 文 献 标 识 码 :f{ 文 章 编 号 :l002 '2872'《2()1 8 【1 【1.{
1 氧 化 锆 陶 瓷 概 述
瓴化 竹 (Zr():、) ·种 埘 离 濉 、蚶 惜 蚀 、耐磨 损
2 氧 化 锆 陶 瓷 制 备 工 艺
尖晶石的三种结构

尖晶石的三种结构尖晶石(Spinel)是一种矿物,化学式为MgAl2O4,属于单斜晶系。
它的晶体结构有三种主要形式,分别是正常尖晶石、反尖晶石和双尖晶石。
下面将详细介绍这三种尖晶石的结构。
1.正常尖晶石结构:正常尖晶石是最常见的尖晶石结构,它的晶体状呈现为立方体或八面体。
正常尖晶石晶体结构具有8个同等位置的离子坐标,其中4个位于正方形的平面上,另外4个位于正方形上下两端。
这种结构中铝离子和镁离子以三维的方式填充晶格。
每个铝离子都被6个氧离子包围,并且每个镁离子也被6个氧离子包围。
这种结构使得正常尖晶石呈现出良好的坚硬性和稳定性。
2.反尖晶石结构:反尖晶石是另一种尖晶石的晶体结构形式。
与正常尖晶石相比,反尖晶石中铝离子和镁离子的位置互换。
在反尖晶石中,铝离子通常占据正常尖晶石中的镁离子位置,而镁离子则占据铝离子的位置。
这种结构的变化导致了晶格的畸变,并且反尖晶石的晶体形状通常是六面体或柱状。
3.双尖晶石结构:双尖晶石是指同时存在正常尖晶石和反尖晶石两种结构的尖晶石矿物。
它的晶体结构与正常尖晶石相似,但其中一些离子位置被铝离子和镁离子的互换所占据。
这种结构导致了晶格的畸变,并且双尖晶石的晶体形状也与正常尖晶石有所不同。
双尖晶石通常呈现出八面体形状,但其中一些面可能会显示出六边形的形状。
总结起来,尖晶石的三种结构包括正常尖晶石、反尖晶石和双尖晶石。
正常尖晶石是最常见的形式,其晶格结构中铝离子和镁离子以三维的方式填充,呈现出立方体或八面体的形状。
反尖晶石与正常尖晶石相比,铝离子和镁离子的位置发生互换,导致晶格的畸变,晶体形状通常为六面体或柱状。
双尖晶石则是同时存在正常尖晶石和反尖晶石两种结构的尖晶石矿物,晶体形状通常为八面体,但可能包含一些六边形面。
这些不同的尖晶石结构不仅对其物理和化学性质产生影响,还使之具备了丰富的应用价值。
- 1、下载文档前请自行甄别文档内容的完整性,平台不提供额外的编辑、内容补充、找答案等附加服务。
- 2、"仅部分预览"的文档,不可在线预览部分如存在完整性等问题,可反馈申请退款(可完整预览的文档不适用该条件!)。
- 3、如文档侵犯您的权益,请联系客服反馈,我们会尽快为您处理(人工客服工作时间:9:00-18:30)。
ORIGINAL PAPERExcellent cycling stability of spherical spinel LiMn2O4by Y2O3 coating for lithium-ion batteriesBowei Ju&Xianyou Wang&Chun Wu&Qiliang Wei&Xiukang Yang&Hongbo Shu&Yansong BaiReceived:28July2013/Revised:21August2013/Accepted:23August2013#Springer-Verlag Berlin Heidelberg2013Abstract The Y2O3nano-film is coated on the surface of the spherical spinel LiMn2O4by precipitation method and subse-quent heat treatment at550°C for5h in air.The structure and performance of the bare LiMn2O4and Y2O3-coated LiMn2O4 are characterized by powder X-ray diffraction,scanning elec-tron microscopy,transmission electron microscopy,energy dispersive analysis X-ray spectroscopy,galvanostatic charge–discharge,cyclic voltammetry,and impedance spec-troscopy.It has been found that the addition of Y2O3does not change the bulk structure of LiMn2O4,and the thickness of the Y2O3coating layer is approximate to3.0nm.The1wt% Y2O3-coated LiMn2O4electrode reveals excellent cycling performance with80.3%capacity retention after500cycles at1C at25°C.When cycling at elevated temperature55°C, the as-prepared sample still shows76.7%capacity retention after500cycles.These remarkable improvements indicate that thin Y2O3coating on the surface of LiMn2O4is an effective way to improve the electrochemistry performance. Besides,the suppression of Mn dissolution into the electrolyte via the Y2O3coating layer can be accounted for the improved performances.Keywords Lithium ion batteries.Spherical spinel lithium manganese oxide.Surface coating.Yttrium oxide.Cycling stabilityIntroductionLithium-ion batteries,which use the lithium transition metal oxides as the positive electrode,have become attractive energy storage systems for portable electronic devices and the powering of emission-free vehicles[1–3].Many studies of lithium rechargeable batteries have been performed in attempts to develop a nontoxic cathode material with a high power density and excellent thermal stability.In this regard, LiMn2O4with a spinel structure is one of the most promising cathode materials due to its good intrinsic characteristics,such as high energy density,low cost,environmental friendliness, and high thermal stability under severe environmental condi-tions[4,5].However,LiMn2O4suffers fast capacity fading during cycling,especially at elevated temperatures,which is the major obstacle to its further commercialization.The rea-sons for capacity fading are ascribed to several factors:Jahn–Teller distortion[6–8],Mn3+dissolution[9],and electrolyte oxidation[10].To overcome the aforementioned problems,two types of methods can be employed.One is the elemental substitution in crystal lattice to stabilize the crystal structure[11–13], although the elemental substitution cathode materials still suffer from significant capacity decline at elevated tempera-ture(50–60°C).The other method is to coat LiMn2O4with various protective layers.Many researchers have attempted to improve the cyclic performance by means of metallic oxide coating layers,which inhibit the Mn3+dissolution of LiMn2O4,such as Al2O3[14–16],SiO2[17],La2O3[18], TiO2[19,20],ZrO2[21,22],CeO2[23–25],MgO[26],and LiAlO2[27].Among these,Arumugam et al.[18]have re-ported that the2.0wt%SiO2-coated LiMn2O4exhibits a good cyclic performance.The capacity retention is95.2%after 100cycles at1at30°C.Another report by Ha et al.[25] describes the improvement of the cycle behavior of2wt% CeO2-coated LiMn2O4,and the capacity retention of the coat-ed LiMn2O4is more than82%after150cycles between3.0 and4.4V at1C at room temperature.Over the past several years,yttria oxide has been used as various ceramics and luminescent materials because of its high corrosionresistance, B.Ju:X.Wang(*):C.Wu:Q.Wei:X.Yang:H.Shu:Y.BaiKey Laboratory of Environmentally Friendly Chemistry andApplications of Ministry of Education,School of Chemistry,Xiangtan University,Xiangtan,Hunan411105,Chinae-mail:wxianyou@J Solid State ElectrochemDOI10.1007/s10008-013-2241-xoutstanding thermostability,and excellent chemical inertness.If these merits could be applied in the regards of lithium ion batteries,it will be a significant work.It will significantly reduce the direct contact area of LiMn 2O 4/electrolyte interface and decrease the dissolution of manganese effectively.Bai et al.[28]coated Y 2O 3on the LiMn 2O 4by mixing Y(NO 3)3with commercial LiMn 2O 4,and the electrochemical perfor-mance of the formless Y 2O 3-coated LiMn 2O 4synthesized via this simple method is relatively limited.Moreover,the analy-sis of the electrochemical properties is also insufficient.In this paper,Y 2O 3have been successfully coated on the surface of spherical LiMn 2O 4sample by precipitation method.The ef-fects of coating on the structural and electrochemical proper-ties are investigated in detail.ExperimentalPreparation of the spherical spinel LiMn 2O 4The precursor MnCO 3is synthesized by the coprecipitation method.An aqueous solution of MnSO 4with a concentration of 1.6mol L −1is pumped into a continuously stirred tank reactor.At the same time,a 1.6mol L −1Na 2CO 3solution (aq)and the desired amount of NH 4OH solution (aq)as the chelating agent are also separately fed into the reactor.The obtained spherical MnCO 3precursor is then filtered,washed,and dried at 80°C.Then,the as-prepared powder and an appropriate amount of Li 2CO 3are mixed homogeneously and calcined at 750°C for 20h to obtain the spherical spinel LiMn 2O 4.Synthesis of the Y 2O 3-coated LiMn 2O 4The Y 2O 3-coated spherical LiMn 2O 4are prepared via precip-itation method.Figure 1shows the schematic diagram ofthe formation process of the Y 2O 3-coated LiMn 2O 4.Y(NO 3)3·6H 2O are dissolved in distilled water,and the pH value is adjusted to about 9.0by aqueous buffer solution of NH 4Cl/NH 3·H 2O with stirring.Subsequently,the as-prepared LiMn 2O 4powders is added to the solution with vigorous stirring for 2h,sonicated for 2h to obtain a suspension,then filtered and washed to obtain a black deposit,and finally evaporated at 80°C.The obtained coated LiMn 2O 4powder is heated at 550°C for 5h.The expected amount of the Y 2O 3coating is 1and 2wt%of the total LiMn 2O 4powder weight,respectively.Physical characterizationsThe phase identification of the samples is performed with a diffractometer (D/Max-3C,Rigaku,Japan)using Cu K αra-diation (λ=1.54178Å)and a graphite monochromatoratFig.1Schematic diagram of the formation process of the Y 2O 3-coated LiMn 2O4Fig.2X-ray diffraction patterns of the bare LiMn 2O 4and Y 2O 3-coated LiMn 2O 4samplesFig.3TG and TGA curves for the Y(OH)3-covered LiMn 2O 4materialsJ Solid State Electrochem36kV ,20mA.The scanning rate is 8°min −1,and the scanning range of diffraction angle (2θ)is 10°≤2θ≤80°.Thermal gravimetric analysis (TG/TGA)of Y(OH)3covered sample is made on a TG-DTA analyzer (Model TAS-200,Rigaku,Tokyo,Japan)in flowing air atmosphere (200ml min −1)with a heating rate of 10°C min −1.The morphology of the sample is observed using scanning electron microscopy (SEM,JEOL JSM-5600LV)and high-resolution transmission electron mi-croscopy (HRTEM,JEOL JEM 2010).Energy dispersive analysis spectroscopy is obtained in conjunction with TEM to roughly determine the element content of powders.Electrochemical characterizationsThe electrochemical tests are examined using CR2025coin-type cells.In all cells,the cathode consisted of a mixture of active material (80wt%),acetylene black (10wt%),graphite (5wt%),and polyvinylidene fluoride (5wt%)as the binderagent;lithium metal is used as the counter and reference electrodes;a Celgard 2400is used as the separator;and the electrolyte is a 1mol L −1LiPF 6solution in ethylene carbon-ate –dimethyl carbonate (1:1,v /v ).The charge –discharge mea-surements are carried out on a Neware battery test system (BTS-XWJ-6.44S-00052;Newell,Shenzhen,China).The electrochemical impedance spectroscopy (EIS)of the cells is measured on a VersaSTAT3electrochemical workstation (Princeton,America)in the frequency range of 10kHz to 10mHz with an AC voltage of 5mV .Results and discussionStructure and morphology analysisFigure 2shows the X-ray diffraction (XRD)patterns of the bare LiMn 2O 4and Y 2O 3-coated LiMn 2O 4samples.All of thea bdc e fFig.4SEM images of a the bare LiMn 2O 4,b 0.5wt%,c 1.0wt%,d 2.0wt%Y 2O 3coated LiMn 2O 4samples at high magnification and e the bare LiMn 2O 4,f 1.0wt%Y 2O 3-coated LiMn 2O 4samples at low magnificationJ Solid State Electrochemdiffraction peaks correspond to a well-defined cubic spinel structure with space group F d 3m except for the low intensity peaks at 2θ=21–24°that assigned to Y 2O 3in 2.0wt%Y 2O 3-coated LiMn 2O 4.The impurity diffraction peaks cannot be observed in 0.5and 1.0wt%Y 2O 3-coated LiMn 2O 4samples.It is possible because the content of Y 2O 3is low and the nanothin film of Y 2O 3on the surface of LiMn 2O 4may be too thin to be detected.Furthermore,the lattice constant of uncoated LiMn 2O 4and 0.5,1.0,and 2.0wt%Y 2O 3-coated LiMn 2O 4samples are0.825,0.824,0.824,and 0.824nm,respectively.Small changes in the lattice parameter for all samples show that Y 2O 3coating does not enter the spinel structure but is just deposited on the surface of LiMn 2O 4.TG/TGA is used to determine the decomposition tempera-ture of the Y(OH)3covered sample.In order to observe clearly weight loss of TG curve and estimate the decomposition temperature,the 50wt%Y(OH)3covered sample are purpose-fully chosen for the measurement.As seen in Fig.3,the first weight loss is seen between 150and 210°C due to thec dba eFig.5TEM images of a the pristine LiMn 2O 4and b 1wt%Y 2O 3-coated LiMn 2O 4,HRTEM images of c the pristine LiMn 2O 4and d 1wt%Y 2O 3-coated LiMn 2O 4,EDAX results of e 1wt%Y 2O 3-coated LiMn 2O 4J Solid State Electrochemevaporation of hydrate from the powder[29].Then,a distinct decrease is seen at500°C,which may be attributed to the decomposition and combustion of yttrium hydroxide and slight yttrium nitrate.The third weight loss in the range of 780–840°C should be related to a partial decomposition of the LiMn2O4to Li–Mn–O compounds containing a higher con-centration of Mn3+[7].However,no obvious change is ob-served at above900°C.Therefore,the heat treatment temper-ature of550°C is chosen for after coating procedure.The surface morphology of the bare LiMn2O4and Y2O3-coated LiMn2O4particles are presented in Fig.4.As shown in Fig.4a,the sample is made up of secondary particles formed by the primary particles(<1μm)aggregating together. Moreover,in Fig.4b–d,the edges and corners of the coated primary particles become blurred and the surfaces of the coated samples become more and more coarse.The reason is due to presence of the Y2O3coating layers,LiMn2O4 particles can prevent from contacting with the electrolyte,thusinhibiting Mn3+dissolution.Furthermore,it can be observed in Fig.4e and f that the particle sizes of bare spinel are uniform,and there are not clear size differences between the bare and the1.0wt%Y2O3coated LiMn2O4particles.To further identify the surface morphology of the bare LiMn2O4and Y2O3-coated LiMn2O4,TEM(HRTEM)and energy dispersive analysis X-ray spectroscopy(EDAX)are car-ried out.The spherical particles are ground by agate mortar before placed on a carbon film-coated TEM grid.Figure5a and b presents the TEM images of the bare LiMn2O4and 1.0wt%Y2O3-coated LiMn2O4cathode materials.The pristine LiMn2O4exhibits smooth surface,while the surface-treated LiMn2O4shows coarse surface and is covered by a uniform nanofilm.It may be caused by the electrochemically inactive Y2O3layer.Examination at higher resolution(Fig.5d)further reveals that the coating layers are uniformly distributed on the particle surfaces and the thickness approximate to2.9nm.It manifests that Y2O3coating has been successfully deposited on the surface of the spherical LiMn2O4.Figure5e shows the EDAX results of1.0wt%Y2O3-coated LiMn2O4.The existence of Y suggests that Y2O3may exist in the surface of the treated LiMn2O4.Electrochemical performanceThe initial charge–discharge curves of the bare LiMn2O4and 0.5,1,and2wt%Y2O3-coated LiMn2O4samples at a rate of 0.5C between3.0and4.4Vat room temperature are shown in Fig.6.All the charge–discharge curves exhibit two obvious discharge plateaus associated with the two-stage mechanism of the electrochemical lithium intercalation and extraction at 3.9–4.0V and4.0–4.1V[30].This indicates that the Y2O3 coating does not change the intrinsic structure of LiMn2O4 during the insertion and extraction of lithium ions.Instead,the surface coating can help to stabilize the structure of LiMn2O4and prevent fast capacity decay,which will be further con-firmed and supported by cyclic voltammetry(CV)curves. Compared with the bare one,the Y2O3-coated LiMn2O4sam-ples show slightly low capacity.It is probably because Y2O3 coating is electrochemically inactive.Moreover,the Y2O3 layer may both increase the contact resistance between parti-cles and the charge transfer resistance between the cathode and the electrolyte.The electrochemical cycling performances of the bare LiMn2O4and the0.5,1,and2wt%Y2O3-coated LiMn2O4at 1C between3.0and4.4V at room temperature are shown in Fig.7.The Y2O3-coated sample shows a much better cycling capability than the uncoated sample.The discharge capacity of the bare LiMn2O4fades from127.4to74.0mA h g−1after 500cycles,58.2%of the original capacity is only reserved. However,the capacity retentions are70.0%for0.5wt%, 80.3%for1.0wt%,and70.2%for2.0wt%Y2O3coating Fig.6Initial charge–discharge curves of the bare and Y2O3-coated LiMn2O4at0.5C in the voltage range of3.0–4.4VFig.7Cycling performances of the bare LiMn2O4and Y2O3-coated LiMn2O4cycle at1C between3.0and4.4V at room temperatureJ Solid State Electrochemafter 500cycles,respectively.These results apparently manifests that the Y 2O 3coating can facilitate the diffusion of lithium ions with the appropriate content.It also can be clearly observed that the cycle performance of the Y 2O 3-coated LiMn 2O 4samples increases with the content of coating increasing from 0.5to 1.0wt%,while it decreases with the content of Y 2O 3coating increasing from 1.0to 2.0wt%.With a too low Y 2O 3coating amount,the LiMn 2O 4surface may not be fully protected,and results in inferior cycling performance.Besides,as Y 2O 3increases beyond the optimal coating con-tent,the excess Y 2O 3will hinder the transportation of lithium ions,leading to the evident decay of the capacity.However,there is not notable decrease in the initial discharge capacity between the bare LiMn 2O 4(127.4mA h g −1)and 1.0wt%Y 2O 3-coated sample (123.9mA h g −1).Based on the above analysis,1.0wt%Y 2O 3-coated LiMn 2O 4is the optimum composition to enhance the stability and cycling performances of LiMn 2O 4.The elevated temperature (55°C)performance is usually an important evaluation criterion for lithium ion battery.At an elevated temperature of 55°C,1.0wt%Y 2O 3-coated mate-rials exhibit distinctly improved capacity retention compared with the pristine LiMn 2O 4.The cycling performances of the pristine LiMn 2O 4and 1.0wt%Y 2O 3-coated LiMn 2O 4sample at 55°C are shown in Fig.8.The discharge capacity of the bare LiMn 2O 4fades from 127.2to 43.0mA h g −1with a retention of 34.1%after 500cycles between 3.0and 4.4V at 1C.However,the discharge capacity of the 1.0wt%Y 2O 3-coated LiMn 2O 4clearly shows an improved cycling be-havior compared with the bare one in the same conditions.The discharge capacity of the 1.0wt%Y 2O 3-coated LiMn 2O 4fades from 117.5to 90.2mA h g −1with a retention of 76.7%of its initial capacity.In addition,this is markedly superior to the results modified by Al 2O 3[14–16],SiO 2[17],La 2O 3[18],TiO 2[19,20],ZrO 2[21,22],CeO 2[23,25],MgO [26],and LiAlO 2[27].Therefore,it is proposed that 1.0wt%Y 2O 3coating is an outstandingly effective way for improving the elevated temperature capacity retention of LiMn 2O 4.In order to investigate the effect of Y 2O 3-coating on the mechanism of spinel LiMn 2O 4,cyclic voltammetry tests are conducted on the pristine LiMn 2O 4and 1.0wt%Y 2O 3-coated sample at a scan rate of 0.1mV s −1in the voltage range of 3.0–4.4Vat 25°C.As seen from Fig.9,two cyclic voltammograms with two couples of well-defined redox peaks and similar shape further reveal that the Y 2O 3coating does not alter the electro-chemical mechanism of LiMn 2O 4during cycling.These two couples of redox peaks reflect the typical redox processes of these electrodes in the potential region from 4.0to 4.2V ,which can be ascribed to electrochemical insertion and extraction reactions of Li +proceed in two steps [31–33].In addition,the CV peaks related to the 1.0wt%Y 2O 3-coated samples are much sharper and appear with much smaller hysteresis,compared to the peaks of the uncoated LiMn 2O 4.These differences mean that the kinetic properties of the Y 2O 3-coat-ed LiMn 2O 4are better than those of uncoated material [19].Fig.9Cyclic voltammetric curves of initial and 500th cycle for a the bare LiMn 2O 4and b 1.0wt%Y 2O 3-coated LiMn 2O 4cycled between 3.0and 4.4V at a scan rate of 0.1mV s −1at roomtemperatureFig.8Cycling performances of the bare LiMn 2O 4and 1.0wt%Y 2O 3-coated LiMn 2O 4cycled between 3.0and 4.4V at elevated temperature (55°C)J Solid State ElectrochemTo further discuss the above results,the CVs of the coated and uncoated samples with the galvanostatic charge –discharge measurements after 500cycles at room temperature are shown in Fig.9.As being noted,the oxidation and reduction peaks related to 1.0wt%Y 2O 3-coated LiMn 2O 4electrode are much steadier compared to the peaks of the bare electrode after 500cycles.Consequently,1.0wt%Y 2O 3-coated LiMn 2O 4has better reversibility and stability than the pristine LiMn 2O 4.In order to further analyze the electrochemical properties about the electrode process and the conductivity of the elec-trode materials,EIS measurements are employed.Figure 10shows the Nyquist plots of the pristine LiMn 2O 4and 1.0wt%Y 2O 3-coated LiMn 2O 4after first,100th cycle and 500th cycle with fully charged state (4.4V)at 1C.The two samples exhibit typical Nyquist characteristics.An intercept in the high frequency region of the Z real axis corresponds to the ohmic resistance (R s ),the combined resistance of the electrolyte and the contacts of the cell [27].The semicircle in high-middle frequency region indicates the charge transfer process on the electrode interface,revealing the lithium transfer rate param-eters as well as the capacitance of the SEI (solid electrolyte interface)/electrolyte double layer [34].The inclined line in the lower frequency region represents the Warburg impedance (Z w )[35],which is associated with the diffusion of Li +in LiMn 2O 4particles.The equivalent circuit used for fitting Nyquist plots is shown in the Fig.10c in which constant phase element stands for the double-layer capacitance.The plots are fitted,and the results are listed in Table 1.The R s for 1.0wt%Y 2O 3-coated LiMn 2O 4exhibits slightly larger than the bare LiMn 2O 4at the first fully charged state.The reason is because the inactive Y 2O 3coating on the sample surface will slightly increase the resistance of the electrolyte and the contacts of thecell before cycling.In the cycling process,the Mn 3+dissolu-tion occurred by the corrosive reaction,which causes the partial electrolyte decomposition and then makes the value of the resistance increased.However,the Y 2O 3coating can inhibit the Mn 3+dissolution and then decrease the tendency of the R s increase.Thus,the R s values of pristine sample became larger than coated sample after cycling.Moreover,the charge transfer resistance (R ct )of the pristine LiMn 2O 4is greatly increased during the cycles.On the contrary,the R ct value of the 1.0wt%Y 2O 3-coated LiMn 2O 4is relatively reduced.The R ct value of the pristine LiMn 2O 4electrode at the first cycle is 25.51and significantly increased to 51.21after 100cycles and 99.37after 500cycles.The coated electrode varied slightly from 26.16at the first cycle to 33.68after 100cycles and 47.64after 500cycles.It means less deposition of Mn and LiF on the electrode surface.Therefore,the enhanced cycling stability of Y 2O 3-coated material should be linked to the improved interfacestability.Fig.10Nyquist plots of a the bare LiMn 2O 4and b 1.0wt%Y 2O 3-coated LiMn 2O 4at 1st,100th,and 500th fully charged state at 1C.c The equivalent circuit modelTable 1The AC impedance fitting results for pristine LiMn 2O 4and 1.0wt%Y 2O 3-coated LiMn 2O 4R s /ΩR ct /ΩPristine LiMn 2O 4at 1st fully charged state2.0325.511.0wt%Y 2O 3-coated LiMn 2O 4at 1st fully charged state 2.1126.16Pristine LiMn 2O 4at 100th fully charged state3.6551.211.0wt%Y 2O 3-coated LiMn 2O 4at 100th fully charged state2.6533.68Pristine LiMn 2O 4at 500th fully charged state5.0199.371.0wt%Y 2O 3-coated LiMn 2O 4at 500th fully charged state3.5247.64J Solid State ElectrochemConclusionsA uniform Y2O3nanofilm has expectedly been coated on the spinel LiMn2O4,and the effects on electrochemical perfor-mance are pared with the pristine LiMn2O4, 1.0wt%Y2O3-coated LiMn2O4exhibits much better cycle performance especially at an elevated temperature(55°C). The capacity retentions of1.0wt%Y2O3-coated LiMn2O4at 1C after500cycles are80.3and76.7%at25and55°C, respectively,while those of pristine LiMn2O4are58.0and 34.1%,respectively.The improved performance of the surface-coated sample is because the Y2O3coating on the surface of LiMn2O4can prevent direct contact between the LiMn2O4particles and electrolyte,thus reducing the dissolu-tion of Mn3+and the oxidation of electrolyte.EIS also shows that the coated sample possesses a lower charge transfer resistance(R ct)than the uncoated sample during cycling. Therefore,it is demonstrated that a uniform,thin Y2O3coating on the surface of LiMn2O4is an effective way to improve the electrochemistry performance.Acknowledgments This work was financially supported by the Na-tional Natural Science Foundation of China under project no.20871101, Joint Fund of Natural Science of Hunan Province and Xiangtan City under project no.09BG005,and Project of Condition Research of Hunan Province under project no.2010TC2004Colleges.References1.Armand M,Tarascon JM(2008)Building better batteries.Nature451:652–6572.Goodenough JB,Kim Y(2010)Challenges for rechargeable Libatteries.Chem Mater22:587–6033.Bruce PG,Scrosati B,Tarascon JM(2008)Nanomaterials for re-chargeable lithium batteries.Angew Chem Int Ed47:2930–2946 4.Thackeray MM,Johnson PJ,Piccootto LAD(1984)Electrochemicalextraction of lithium from LiMn2O4.Mater Res Bull19:179–187 5.Deng B,Nakamurab H,Yoshiob M(2005)Comparison and im-provement of the high rate performance of different types of LiMn2O4spinels.J Power Sources141:116–1216.Jang DH,Shin YJ,Oh SM(1996)Dissolution of spinel oxides andcapacity losses in4V Li/Li x Mn2O4Cells.J Electrochem Soc143: 2204–22117.Aoshima T,Okahara K,Kiyohara C,Shizuka K(2001)Mechanismsof manganese spinels dissolution and capacity fade at high tempera-ture.J Power Sources97–98:377–3808.Yamada A,Tanaka M,Tanaka K,Sekai K(1999)Jahn–Teller insta-bility in spinel Li–Mn–O.J Power Sources81–82:73–789.Lee SW,Kim KS,Moon HS,Lee JP,Kim HJ,Cho BW,Cho W,ParkJW(2004)Electrochemical and structural characteristics of metal oxide-coated lithium manganese oxide(spinel type):Part I.In the range of2.5-4.2V.J Power Sources130:227–23210.Gao Y,Dahn JR(1996)Correlation between the growth of the3.3Vdischarge plateau and capacity fading in Li1+x Mn2−x O4materials.Solid State Ionics84:33–4011.Zhou F,Zhao XM,Dahn JR(2009)Synthesis,electrochemical prop-erties,and thermal stability of Al-doped LiNi1/3Mn1/3Co(1/3−z)Al z O2 positive electrode materials.J Electrochem Soc156:343–34712.Sigala C,Guyomard D,Verbaere A,Piffard Y,Tournoux M(1995)Positive electrode materials with high operating voltage for lithium batteries:LiCr y Mn(2−y)O4(0<y<1).Solid State Ionics81:167–171 13.Takahashi M,Yoshida T,Ichikawa A,Kitoh K,Katsukawa H,ZhangQ,Yoshio M(2006)Effect of oxygen deficiency reduction in Mg-doped Mn-spinel on its cell storage performance at high temperature.Electrochim Acta51:5508–551414.Lee SW,Kima KS,Moona HS,Kimb HJ,Chob BW,Chob W,Ju JB,Park JW(2004)Electrochemical characteristics of Al2O3-coated lithium manganese pinel as a cathode material for a lithium secondary battery.J Power Sources126:150–15515.Xiang JF,Chang CX,Yuan LJ,Sun JT(2008)A simple and effectivestrategy to synthesize Al2O3-coated LiNi0.8Co0.2O2cathode materials for lithium ion battery.Electrochem Commun10:1360–136316.Kim WK,Han DW,Ryu WH,Lim SJ,Kwon HS(2012)Al2O3coating on LiMn2O4by electrostatic attraction forces and its effects on the high temperature cyclic performance.Electrochim Acta71: 17–2117.Arumugam D,Kalaignan GP(2008)Synthesis and electrochem-ical characterizations of nano-SiO2-coated LiMn2O4cathode ma-terials for rechargeable lithium batteries.J Electroanal Chem624: 197–20418.Arumugam D,Kalaignan GP(2010)Synthesis and electrochemicalcharacterizations of nano-La2O3-coated nanostructure LiMn2O4cath-ode materials for rechargeable lithium batteries.Mater Res Bull45: 1825–183119.Wu F,Wang M,Su YF,Chen S,Xua B(2009)Effect of TiO2-coatingon the electrochemical performances of LiCo1/3Ni1/3Mn1/3O2.J Power Sources191:628–632i C,Wy Y,Liu HY,Wang WJ(2009)Preparation of TiO2-coatedLiMn2O4by carrier transfer method.Ionics15:389–39221.Walz KA,Johnson CS,Genthe J,Stoiber LC,Zeltner W A,AndersonMA,Thackeray MM(2010)Elevated temperature cycling stability and electrochemical impedance of LiMn2O4cathodes with nanoporous ZrO2and TiO2coatings.J Power Sources195:4943–495122.Lim SH,Cho J(2008)PVP-Assisted ZrO2coating on LiMn2O2spinel cathode nanoparticle sprepared by MnO2nanowire templates.Electrochem Commun10:1478–148123.Cho MY,Roh KC,Park SM,Lee JW(2011)Effects of CeO2coatinguniformity on high temperature cycle life performance of LiMn2O4.Mater Lett65:2011–201424.Arumugam D,Kalaignan GP(2010)Synthesis and electrochemicalcharacterization of nano-CeO2-coated nanostructure LiMn2O4cath-ode materials for rechargeable lithium batteries.Electrochim Acta55: 8709–871625.Ha HW,Yun NJ,Kim K(2007)Improvement of electrochemicalstability of LiMn2O4by CeO2coating for lithium-ion batteries.Electrochim Acta52:3236–324126.Gnanaraj JS,Pol VG,Gedanken A,Aurbach D(2003)Improving thehigh-temperature performance of LiMn2O4spinel electrodes by coat-ing the active mass with MgO via a sonochemical method.Electrochem Commun5:940–94527.Jang SB,Kang SH,Amine K,Bae YC,Sun YK(2005)Synthesis andimproved electrochemical performance of Al(OH)3-coated Li[Ni1/3Mn1/ 3Co1/3]O2cathode materials at elevated temperature.Electrochim Acta 50:4168–417328.Bai Y,Wu F,Yang H-T,Zhong Y,Wu C(2012)Surface modificationof spinel LiMn2O4with Y2O3for lithium-ion battery.Adv Mater Res 391–392:1069–107429.Şahan H,Göktepe H,PatatŞ,Ülgen A(2010)Effect of the Cr2O3coating on electrochemical properties of spinel LiMn2O4as acathode material for lithium battery applications.Solid State Ionics181: 1437–144430.Zhou WJ,Bao SJ,He BL,Liang YY,Li HL(2006)Synthesis andelectrochemical properties of LiAl0.05Mn1.95O4by the ultrasonic assisted rheological phase method.Electrochim Acta51:4701–4708J Solid State Electrochem。