Optical interconnect roadmap challenges and critical directions
多尺度上采样方法的轻量级图像超分辨率重建
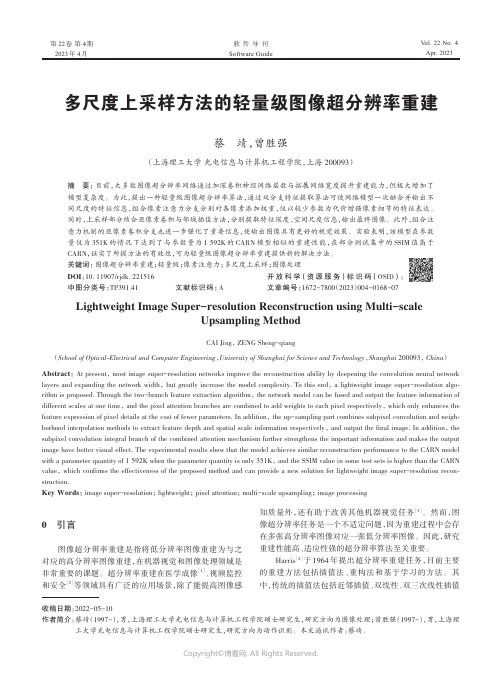
第 22卷第 4期2023年 4月Vol.22 No.4Apr.2023软件导刊Software Guide多尺度上采样方法的轻量级图像超分辨率重建蔡靖,曾胜强(上海理工大学光电信息与计算机工程学院,上海 200093)摘要:目前,大多数图像超分辨率网络通过加深卷积神经网络层数与拓展网络宽度提升重建能力,但极大增加了模型复杂度。
为此,提出一种轻量级图像超分辨率算法,通过双分支特征提取算法可使网络模型一次融合并输出不同尺度的特征信息,组合像素注意力分支分别对各像素添加权重,仅以较少参数为代价增强像素细节的特征表达。
同时,上采样部分结合亚像素卷积与邻域插值方法,分别提取特征深度、空间尺度信息,输出最终图像。
此外,组合注意力机制的亚像素卷积分支也进一步强化了重要信息,使输出图像具有更好的视觉效果。
实验表明,该模型在参数量仅为351K的情况下达到了与参数量为1 592K的CARN模型相似的重建性能,在部分测试集中的SSIM值高于CARN,证实了所提方法的有效性,可为轻量级图像超分辨率重建提供新的解决方法。
关键词:图像超分辨率重建;轻量级;像素注意力;多尺度上采样;图像处理DOI:10.11907/rjdk.221516开放科学(资源服务)标识码(OSID):中图分类号:TP391.41 文献标识码:A文章编号:1672-7800(2023)004-0168-07Lightweight Image Super-resolution Reconstruction using Multi-scaleUpsampling MethodCAI Jing, ZENG Sheng-qiang(School of Optical-Electrical and Computer Engineering,University of Shanghai for Science and Technology,Shanghai 200093, China)Abstract:At present, most image super-resolution networks improve the reconstruction ability by deepening the convolution neural network layers and expanding the network width, but greatly increase the model complexity. To this end, a lightweight image super-resolution algo‐rithm is proposed. Through the two-branch feature extraction algorithm, the network model can be fused and output the feature information of different scales at one time, and the pixel attention branches are combined to add weights to each pixel respectively, which only enhances the feature expression of pixel details at the cost of fewer parameters. In addition, the up-sampling part combines subpixel convolution and neigh‐borhood interpolation methods to extract feature depth and spatial scale information respectively, and output the final image. In addition, the subpixel convolution integral branch of the combined attention mechanism further strengthens the important information and makes the output image have better visual effect. The experimental results show that the model achieves similar reconstruction performance to the CARN model with a parameter quantity of 1 592K when the parameter quantity is only 351K, and the SSIM value in some test sets is higher than the CARN value, which confirms the effectiveness of the proposed method and can provide a new solution for lightweight image super-resolution recon‐struction.Key Words:image super-resolution; lightweight; pixel attention; multi-scale upsampling; image processing0 引言图像超分辨率重建是指将低分辨率图像重建为与之对应的高分辨率图像重建,在机器视觉和图像处理领域是非常重要的课题。
在骨干光网中ROADM技术应用探讨
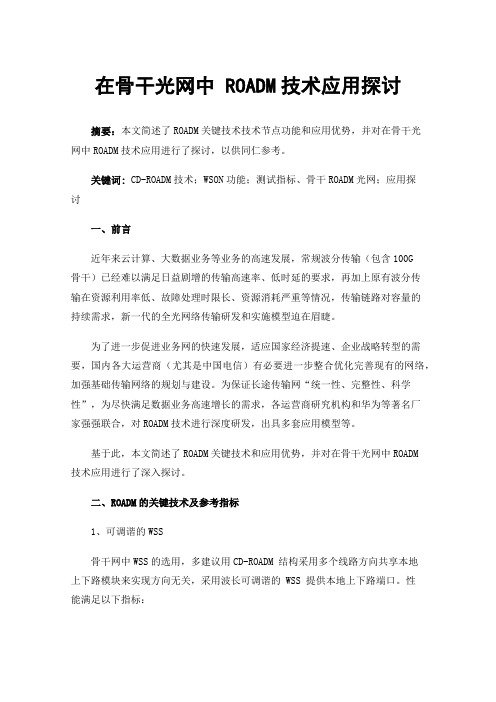
在骨干光网中 ROADM技术应用探讨摘要:本文简述了ROADM关键技术技术节点功能和应用优势,并对在骨干光网中ROADM技术应用进行了探讨,以供同仁参考。
关键词: CD-ROADM技术;WSON功能;测试指标、骨干ROADM光网;应用探讨一、前言近年来云计算、大数据业务等业务的高速发展,常规波分传输(包含100G骨干)已经难以满足日益剧增的传输高速率、低时延的要求,再加上原有波分传输在资源利用率低、故障处理时限长、资源消耗严重等情况,传输链路对容量的持续需求,新一代的全光网络传输研发和实施模型迫在眉睫。
为了进一步促进业务网的快速发展,适应国家经济提速、企业战略转型的需要,国内各大运营商(尤其是中国电信)有必要进一步整合优化完善现有的网络,加强基础传输网络的规划与建设。
为保证长途传输网“统一性、完整性、科学性”,为尽快满足数据业务高速增长的需求,各运营商研究机构和华为等著名厂家强强联合,对ROADM技术进行深度研发,出具多套应用模型等。
基于此,本文简述了ROADM关键技术和应用优势,并对在骨干光网中ROADM技术应用进行了深入探讨。
二、ROADM的关键技术及参考指标1、可调谐的WSS骨干网中WSS的选用,多建议用CD-ROADM 结构采用多个线路方向共享本地上下路模块来实现方向无关,采用波长可调谐的 WSS 提供本地上下路端口。
性能满足以下指标:对WSS的测试主要在工作波长范围、通道间隔、通道带宽、插损及通道间插损不平坦度、通路隔离度、VOA衰减范围和步长等方面进行测试。
2、对光路的需求光放段设置时光纤衰减按测试值计取,同时考虑一定的光缆富余度。
光缆富余度的取定如下(L为光缆长度):当L<=75km时,余量取3dB;当75km余量取(L*0.04)dB;当 L>125km,余量取 5dB。
各光放段光纤衰减值及衰耗富余。
3、误码性能指标在测试时,按照WDM 传输系统配置图图示的光复用段,抽测一个光复用段一个波道的短期误码性能,测试时间为 24 小时,误码指标 ES、SES 应均为0 或无丢包,其余光复用段/通道测试 15 分钟应无误码。
EV-Globe3.0正式发布用户大会圆满闭幕
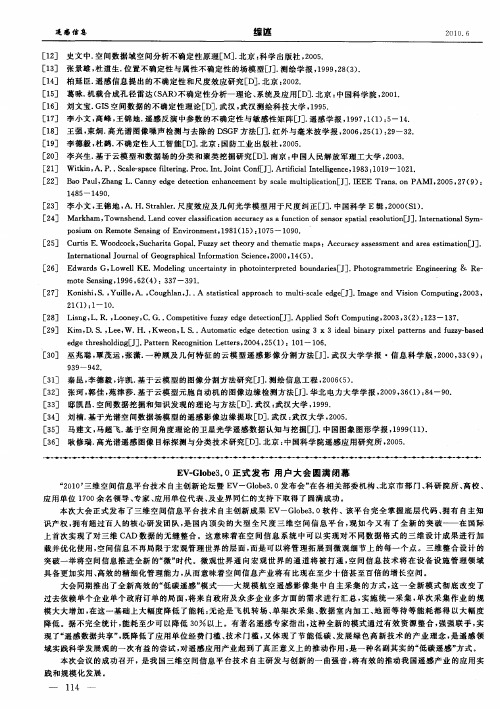
C rsE Wo do k S c ai o a. uz t hoya dte t p : c rc ssmet n ra si t n J . ut . o cc ,uh raC p1F zys er n mai mas Acuaya es n dae t i l] i t - et h c s a e ma o ,
2 () 1 1 . 1 1 : — O
La g L R , o ny C G . o eiv zyeg eet n J. p l dS f C mp t g 2 0 ,() 1 3 3 . i , . .L o e , . . C mpt i f z d edtci [] A pi ot o ui ,0 3 3 2 :2 —1 7 n te u o e n
e g rsodn [] P t r eo nt nL t r,0 42 () 0 — 1 6 d et ehlig J. at nR cg io et s2 0 ,5 1 :1 1 0 . h e i e
巫 兆 聪 , 茂 运 , 潇 . 种 顾 及 几 何 特 征 的 云 模 型遥 感 影 像 分 割 方 法 [] 武 汉 大 学 学 报 ・ 息科 学 版 ,0 0 3 ( ) 覃 张 一 J. 信 20 ,3 9 :
葛 咏 . 载合 成 孔 径 雷 达 (AR 不 确 定 性 分 析一 理 论 、 统 及应 用 [ ] 北 京 : 国科 学 院 ,0 1 机 S ) 系 D. 中 20. 刘 文 宝. I 间 数 据 的不 确 定 性 理 论 [ ] 武 汉 , 汉 测 绘 科 技 大学 ,9 5 G S空 D. 武 19 . 李 小 文 , 峰 , 锦 地. 感 反演 中参 数 的不 确 定 性 与 敏感 性 矩 阵 [] 遥 感 学报 ,9 7 11 :— 1. 高 王 遥 J. 19 ,( ) 5 4
计算机术语英文缩写解释
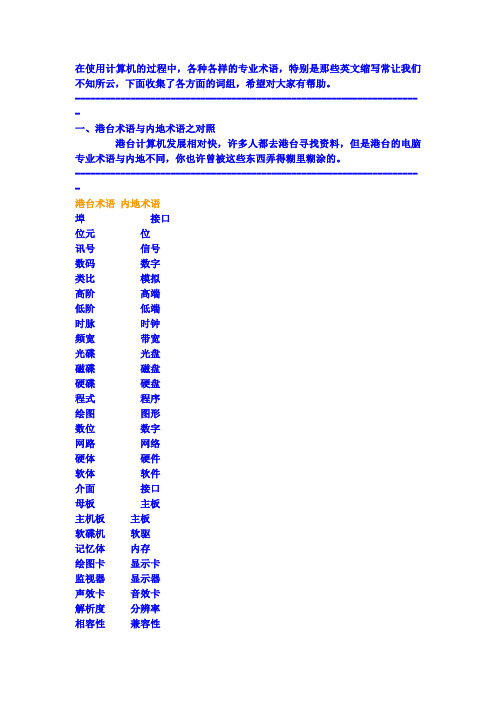
在使用计算机的过程中,各种各样的专业术语,特别是那些英文缩写常让我们不知所云,下面收集了各方面的词组,希望对大家有帮助。
---------------------------------------------------------------------一、港台术语与内地术语之对照港台计算机发展相对快,许多人都去港台寻找资料,但是港台的电脑专业术语与内地不同,你也许曾被这些东西弄得糊里糊涂的。
---------------------------------------------------------------------港台术语内地术语埠接口位元位讯号信号数码数字类比模拟高阶高端低阶低端时脉时钟频宽带宽光碟光盘磁碟磁盘硬碟硬盘程式程序绘图图形数位数字网路网络硬体硬件软体软件介面接口母板主板主机板主板软碟机软驱记忆体内存绘图卡显示卡监视器显示器声效卡音效卡解析度分辨率相容性兼容性数据机调制解调器---------------------------------------------------------------------二、英文术语完全介绍按照字母排序:---------------------------------------------------------------------1、CPU3DNow!(3D no waiting,无须等待的3D处理)AAM(AMD Analyst Meeting,AMD分析家会议)ABP(Advanced Branch Prediction,高级分支预测)ACG(Aggressive Clock Gating,主动时钟选择)AIS(Alternate Instruction Set,交替指令集)ALAT(advanced load table,高级载入表)ALU(Arithmetic Logic Unit,算术逻辑单元)Aluminum(铝)AGU(Address Generation Units,地址产成单元)APC(Advanced Power Control,高级能源控制)APIC(Advanced rogrammable Interrupt Controller,高级可编程中断控制器)APS(Alternate Phase Shifting,交替相位跳转)ASB(Advanced System Buffering,高级系统缓冲)ATC(Advanced Transfer Cache,高级转移缓存)ATD(Assembly Technology Development,装配技术发展)BBUL(Bumpless Build-Up Layer,内建非凹凸层)BGA(Ball Grid Array,球状网阵排列)BHT(branch prediction table,分支预测表)Bops(Billion Operations Per Second,10亿操作秒)BPU(Branch Processing Unit,分支处理单元)BP(Brach Pediction,分支预测)BSP(Boot Strap Processor,启动捆绑处理器)BTAC(Branch Target Address Calculator,分支目标寻址计算器)CBGA (Ceramic Ball Grid Array,陶瓷球状网阵排列)CDIP (Ceramic Dual-In-Line,陶瓷双重直线)Center Processing Unit Utilization,中央处理器占用率CFM(cubic feet per minute,立方英尺秒)CMT(course-grained multithreading,过程消除多线程)CMOS(Complementary Metal Oxide Semiconductor,互补金属氧化物半导体)CMOV(conditional move instruction,条件移动指令)CISC(Complex Instruction Set Computing,复杂指令集计算机)CLK(Clock Cycle,时钟周期)CMP(on-chip multiprocessor,片内多重处理)CMS(Code Morphing Software,代码变形软件)co-CPU(cooperative CPU,协处理器)COB(Cache on board,板上集成缓存,做在CPU卡上的二级缓存,通常是内核的一半速度))COD(Cache on Die,芯片内核集成缓存)Copper(铜)CPGA(Ceramic Pin Grid Array,陶瓷针型栅格阵列)CPI(cycles per instruction,周期指令)CPLD(Complex Programmable Logic Device,複雜可程式化邏輯元件)CPU(Center Processing Unit,中央处理器)CRT(Cooperative Redundant Threads,协同多余线程)CSP(Chip Scale Package,芯片比例封装)CXT(Chooper eXTend,增强形K6-2内核,即K6-3)Data Forwarding(数据前送)dB(decibel,分贝)DCLK(Dot Clock,点时钟)DCT(DRAM Controller,DRAM控制器)DDT(Dynamic Deferred Transaction,动态延期处理)Decode(指令解码)DIB(Dual Independent Bus,双重独立总线)DMT(Dynamic Multithreading Architecture,动态多线程结构)DP(Dual Processor,双处理器)DSM(Dedicated Stack Manager,专门堆栈管理)DSMT(Dynamic Simultaneous Multithreading,动态同步多线程)DST(Depleted Substrate Transistor,衰竭型底层晶体管)DTV(Dual Threshold Voltage,双重极限电压)DUV(Deep Ultra-Violet,纵深紫外光)EBGA(Enhanced Ball Grid Array,增强形球状网阵排列)EBL(electron beam lithography,电子束平版印刷)EC(Embedded Controller,嵌入式控制器)EDB(Execute Disable Bit,执行禁止位)EDEC(Early Decode,早期解码)Embedded Chips(嵌入式)EM64T(Extended Memory 64 Technology,扩展内存64技术)EPA(edge pin array,边缘针脚阵列)EPF(Embedded Processor Forum,嵌入式处理器论坛)EPL(electron projection lithography,电子发射平版印刷)EPM(Enhanced Power Management,增强形能源管理)EPIC(explicitly parallel instruction code,并行指令代码)EUV(Extreme Ultra Violet,紫外光)EUV(extreme ultraviolet lithography,极端紫外平版印刷)FADD(Floationg Point Addition,浮点加)FBGA(Fine-Pitch Ball Grid Array,精细倾斜球状网阵包装)FBGA(flipchip BGA,轻型芯片BGA)FC-BGA(Flip-Chip Ball Grid Array,翻转芯片球形网阵包装)FC-LGA(Flip-Chip Land Grid Array,翻转接点网阵包装)FC-PGA(Flip-Chip Pin Grid Array,翻转芯片球状网阵包装)FDIV(Floationg Point Divide,浮点除)FEMMS:Fast EntryExit Multimedia State,快速进入退出多媒体状态FFT(fast Fourier transform,快速热欧姆转换)FGM(Fine-Grained Multithreading,高级多线程)FID(FID:Frequency identify,频率鉴别号码)FIFO(First Input First Output,先入先出队列)FISC(Fast Instruction Set Computer,快速指令集计算机)flip-chip(芯片反转)FLOPs(Floating Point Operations Per Second,浮点操作秒)FMT(fine-grained multithreading,纯消除多线程)FMUL(Floationg Point Multiplication,浮点乘)FPRs(floating-point registers,浮点寄存器)FPU(Float Point Unit,浮点运算单元)FSUB(Floationg Point Subtraction,浮点减)GFD(Gold finger Device,金手指超频设备)GHC(Global History Counter,通用历史计数器)GTL(Gunning Transceiver Logic,射电收发逻辑电路)GVPP(Generic Visual Perception Processor,常规视觉处理器)HL-PBGA 表面黏著,高耐热、轻薄型塑胶球状网阵封装HTT(Hyper-Threading Technology,超级线程技术)Hz(hertz,赫兹,频率单位)IA(Intel Architecture,英特尔架构)IAA(Intel Application Accelerator,英特尔应用程序加速器)IATM(Intel Advanced Thermal Manager,英特尔高级热量管理指令集)ICU(Instruction Control Unit,指令控制单元)ID(identify,鉴别号码)IDF(Intel Developer Forum,英特尔开发者论坛)IDMB(Intel Digital Media Boost,英特尔数字媒体推进指令集)IDPC(Intel Dynamic Power Coordination,英特尔动态能源调和指令集)IEU(Integer Execution Units,整数执行单元)IHS(Integrated Heat Spreader,完整热量扩展)ILP(Instruction Level Parallelism,指令级平行运算)IMM Intel Mobile Module, 英特尔移动模块Instructions Cache,指令缓存Instruction Coloring(指令分类)IOPs(Integer Operations Per Second,整数操作秒)IPC(Instructions Per Clock Cycle,指令时钟周期)ISA(instruction set architecture,指令集架构)ISD(inbuilt speed-throttling device,内藏速度控制设备)ITC(Instruction Trace Cache,指令追踪缓存)ITRS(International Technology Roadmap for Semiconductors,国际半导体技术发展蓝图)KNI(Katmai New Instructions,Katmai新指令集,即SSE)Latency(潜伏期)LDT(Lightning Data Transport,闪电数据传输总线)LFU(Legacy Function Unit,传统功能单元)LGA(land grid array,接点栅格阵列)LN2(Liquid Nitrogen,液氮)Local Interconnect(局域互连)MAC(multiply-accumulate,累积乘法)mBGA (Micro Ball Grid Array,微型球状网阵排列)nm(namometer,十亿分之一米毫微米)MCA(Machine Check Architecture,机器检查架构)MCU(Micro-Controller Unit,微控制器单元)MCT(Memory Controller,内存控制器)MESI(Modified, Exclusive, Shared, Invalid:修改、排除、共享、废弃)MF(MicroOps Fusion,微指令合并)mm(micron metric,微米)MMX(MultiMedia Extensions,多媒体扩展指令集)MMU(Multimedia Unit,多媒体单元)MMU(Memory Management Unit,内存管理单元)MN(model numbers,型号数字)MFLOPS(Million Floationg PointSecond,每秒百万个浮点操作)MHz(megahertz,兆赫)mil(PCB 或晶片佈局的長度單位,1 mil = 千分之一英寸)MIMD(Multi Instruction Multiple Data,多指令多数据流)MIPS(Million Instruction Per Second,百万条指令秒)MOESI(Modified, Owned, Exclusive, Shared or Invalid,修改、自有、排除、共享或无效)MOF(Micro Ops Fusion,微操作熔合)Mops(Million Operations Per Second,百万次操作秒)MP(Multi-Processing,多重处理器架构)MPF(Micro processor Forum,微处理器论坛)MPU(Microprocessor Unit,微处理器)MPS(MultiProcessor Specification,多重处理器规范)MSRs(Model-Specific Registers,特别模块寄存器)MSV(Multiprocessor Specification Version,多处理器规范版本)MVP(Mobile Voltage Positioning,移动电压定位)IVNAOC(no-account OverClock,无效超频)NI(Non-Intel,非英特尔)NOP(no operation,非操作指令)NRE(Non-Recurring Engineering charge,非重複性工程費用)OBGA(Organic Ball Grid Arral,有机球状网阵排列)OCPL(Off Center Parting Line,远离中心部分线队列)OLGA(Organic Land Grid Array,有机平面网阵包装)OoO(Out of Order,乱序执行)OPC(Optical Proximity Correction,光学临近修正)OPGA(Organic Pin Grid Array,有机塑料针型栅格阵列)OPN(Ordering Part Number,分类零件号码)PAT(Performance Acceleration Technology,性能加速技术)PBGA(Plastic Pin Ball Grid Array,塑胶球状网阵排列)PDIP (Plastic Dual-In-Line,塑料双重直线)PDP(Parallel Data Processing,并行数据处理)PGA(Pin-Grid Array,引脚网格阵列),耗电大PLCC (Plastic Leaded Chip Carriers,塑料行间芯片运载)Post-RISC(加速RISC,或后RISC)PPE(Power Processor Element,Power处理器元件)PPU(Physics Processing Unit,物理处理单元)PR(Performance Rate,性能比率)PIB(Processor In a Box,盒装处理器)PM(Pseudo-Multithreading,假多线程)PPGA(Plastic Pin Grid Array,塑胶针状网阵封装)PQFP(Plastic Quad Flat Package,塑料方块平面封装)PSN(Processor Serial numbers,处理器序列号)QFP(Quad Flat Package,方块平面封装)QSPS(Quick Start Power State,快速启动能源状态)RAS(Return Address Stack,返回地址堆栈)RAW(Read after Write,写后读)REE(Rapid Execution Engine,快速执行引擎)Register Contention(抢占寄存器)Register Pressure(寄存器不足)Register Renaming(寄存器重命名)Remark(芯片频率重标识)Resource contention(资源冲突)Retirement(指令引退)RISC(Reduced Instruction Set Computing,精简指令集计算机)ROB(Re-Order Buffer,重排序缓冲区)RSE(register stack engine,寄存器堆栈引擎)RTL(Register Transfer Level,暫存器轉換層。
adas面试问题
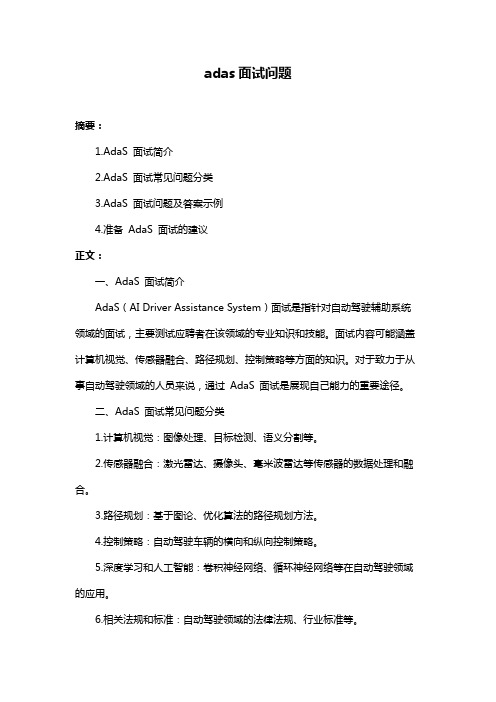
adas面试问题摘要:1.AdaS 面试简介2.AdaS 面试常见问题分类3.AdaS 面试问题及答案示例4.准备AdaS 面试的建议正文:一、AdaS 面试简介AdaS(AI Driver Assistance System)面试是指针对自动驾驶辅助系统领域的面试,主要测试应聘者在该领域的专业知识和技能。
面试内容可能涵盖计算机视觉、传感器融合、路径规划、控制策略等方面的知识。
对于致力于从事自动驾驶领域的人员来说,通过AdaS 面试是展现自己能力的重要途径。
二、AdaS 面试常见问题分类1.计算机视觉:图像处理、目标检测、语义分割等。
2.传感器融合:激光雷达、摄像头、毫米波雷达等传感器的数据处理和融合。
3.路径规划:基于图论、优化算法的路径规划方法。
4.控制策略:自动驾驶车辆的横向和纵向控制策略。
5.深度学习和人工智能:卷积神经网络、循环神经网络等在自动驾驶领域的应用。
6.相关法规和标准:自动驾驶领域的法律法规、行业标准等。
三、AdaS 面试问题及答案示例1.问题:简述计算机视觉在自动驾驶中的应用。
答案:计算机视觉在自动驾驶中的应用主要包括:(1)环境感知:通过图像处理技术识别道路、车辆、行人等物体,为自动驾驶提供环境信息。
(2)目标检测:实时检测道路中的障碍物、车辆、行人等,为自动驾驶提供目标信息。
(3)语义分割:对道路场景进行分割,提取道路、车辆、行人等区域,辅助自动驾驶决策。
2.问题:介绍一下激光雷达的工作原理。
答案:激光雷达通过发射激光束,测量返回激光束的时间差来计算目标距离。
其工作原理主要包括:(1)发射:激光器发出激光束。
(2)接收:接收器接收反射回来的激光束。
(3)计时:记录激光往返时间,计算目标距离。
(4)数据处理:根据距离信息生成点云数据,用于后续处理。
四、准备AdaS 面试的建议1.扎实的基础知识:掌握计算机视觉、传感器融合、路径规划、控制策略等基础知识。
2.实践经验:积累实际项目经验,了解自动驾驶领域的技术应用。
富士通 FLASHWAVE
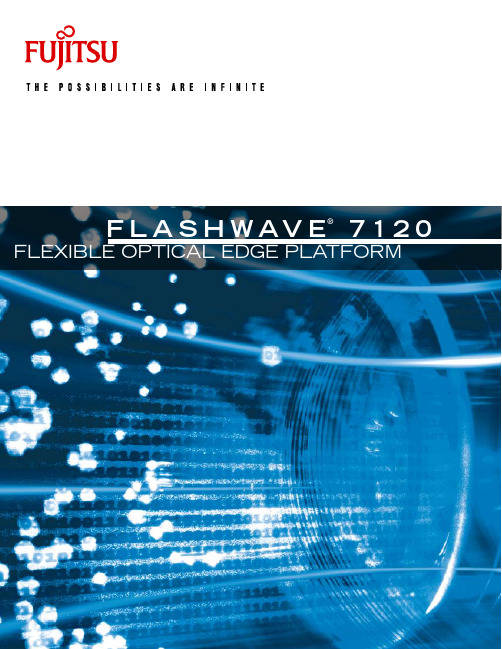
F L A S H W A V E®7120 FLEXIBLE OPTICAL EDGE PLA TFORMWavelength service customers are dispersed over larger geographic areas than ever before. They demand an unpredictable mix of voice, data and video at competitive prices. Consequently, carriers are under pressure to deliver advanced services beyond the typical reach of metro optics or available fiber, while aggressivelymanaging expenses. To succeed, service providers must extend DWDM and CWDM services with infrastructure that adapts to emerging bandwidth and access-backhaul demands.Reduced Complexity, Extended ReachThe FLASHWAVE ® 7120 optical edge platform greatly reduces the operational complexity of adding incremental bandwidth and functionality. It combines multiplexing, amplification, signal conditioning and performance monitoring in a powerful, customized solution that is easy to deploy in metro and metro access networks. The platform meets optical transport, conditioning and extension requirements with a small footprint.The FLASHWAVE 7120 system operates as a standalone system or as an extension of an existing transport infrastructure. It provides simplicity and efficiency in a compact and flexible platform that addresses multiple network challenges, including:Optical metro edge networking ▶Wavelength service delivery ▶Fiber relief▶Optical reach extension▶Trusted to DeliverFujitsu is a trusted business partner of major carriers in North America. For more than 20 years, we’ve set the standard for performance, reliability and carrier-grade quality. The FLASHWAVE 7120 platform is an ideal solution for national and local carriers, wireless service providers, research and education, utility companies and enterprise customers.ADAPT TO EMERGING DEMANDLOCAL CARRIERSUTILITY COMPANIESWIRELESS SERVICEPROVIDERSNATIONAL CARRIERSRESEARCH &EDUCATION FACILITIESENTERPRISE NETWORKSCONNECT, EXTEND, EXPANDThe unique FLASHWAVE 7120 photonic layer solution combines the reliability and management of traditional optical transport systems with flexibility and cost-efficiency.Its comprehensive suite of passive and active modules permits highly flexible customization. This facilitates control of capital outlay by mixing and matching components for unique requirements and immediateequipment needs. As a result, you reach more customers at a lower cost.Options for Different Network RequirementsServices can be directly interfaced into the FLASHWAVE 7120 platform through its full suite of muxponders and transponders. The system’s compact, 2RU shelf supports:▶Full suite of muxponders and transponders including:•Dual transpondersHigh-density muxponders•Tunable optics••SFP/XFP-based client and network optionsDigital wrapper support•Optimized signal conditioning and amplification▶DWDM and CWDM filters▶S ingle controller with integrated communications (OSC) for point-to-point or ring applications ▶▶Active and passive-only applications4-shelf (8RU) stacking for a full 32 x 10G channel configuration with in-service channel additions ▶Multiservice Overlay SupportBy combining multiprotocol/SONET connectivity with OTN and packet-based transport, the platform isideal for multiservice overlay installations that facilitate data, storage and legacy network convergence. The FLASHWAVE 7120 system supports up to 20 multiservice channels in a compact 2RU chassis. It utilizes existing fiber without requiring changes to SONET connectivity. A multiprotocol muxponder provides a high-density interface to a wide range of protocols used in enterprise and service provider networks to maximize DWDMThe FLASHWAVE 7120 platform delivers cost-optimized amplification, signal conditioning and optical/electrical switching. It offers easy scalability in addition to advanced optical technologies such as full-band tunables and OTN transport. The platform also offers simplified operations, administration and provisioning with software download and remote memory backup/restore.Manageable FlexibilityThe FLASHWAVE 7120 architecture scales so that service slots from multiple shelves can be managed from a single CPU and Target Identifier (TID). The NETSMART ® 500 craft user interface and NETSMART 1500 Element Management System (EMS), via simple smart launch, allow the FLASHWAVE 7120 platform to be managed under the same OSS as other Fujitsu optical transport products. Transaction Language 1 (TL1) and Simple Network Management Protocol (SNMP) support enable the platform to integrate into existing operating procedures and third-party management systems.Reduce Demand on Your InfrastructureWhen combined with multiple narrowband wavelengths from the FLASHWAVE 4500 Multiservice Provisioning Platform (MSPP) or FLASHWAVE 7500 Extension System, the resulting single DWDM signal reduces the demand on your fiber infrastructure.Key Technical AdvantagesBy combining multiplexing, amplification, signal conditioning, optical add/drop and performance monitoring, the FLASHWAVE 7120 system delivers several technical advantages.REACH MORE CUSTOMERSKEY NETWORK BENEFITSKEY TECHNICAL ADVANTAGESSEAMLESS INTEGRATIONLOWER COSTSREDUCE CIRCUIT ACTIVATION TIMEEFFICIENT BANDWIDTH GROWTHSIMPLE NETWORK MANAGEMENTDENSE GIGABITETHERNET MULTIPLEXINGEFFICIENT SONET/SDHMULTIPLEXINGINTEGRATED OPTICAL LAYER FUNCTIONSCOMPLETE OTN SUPPORTCOMPACT WDM TRANSPORTCRITICAL NETWORK APPLICAOptical Extension and Fiber ReliefThe FLASHWAVE 7120 platform offers flexible and powerful optical capabilities for cost-effective, small-footprint extension of wavelength services and access service backhaul:IP-DSLAM and WiMAX backhaul▶Legacy service CWDM and DWDM overlay▶Regeneration or MSPP/ADM site elimination▶Access fiber relief▶▶Transparent network interconnection with OC-192/STM-64 or OTN digital wrapperWavelength Service DeliveryThe FLASHWAVE 7120 platform provides advanced optical technologies for WDM (DWDM and CWDM) and multiservice customer needs:Managed Ethernet private-line service delivery▶Ethernet business service delivery (E-LAN, E-TREE)▶DSL backhaul▶Sub-wavelength wholesale Ethernet interconnection▶Managed private-line service delivery▶Ethernet, Storage Area Networks, SONET and SDH▶Enterprise and Dedicated Customer Carrier NetworksExceptional entry price, small footprint, remote management and SNMP make this CPE-capable system perfect for dedicated network installations:PON▶IPTV distribution▶10 Gbps Ethernet rings▶Interoffice router connectivity▶▶High-capacity LAN extension/routes and switch connectivityData center interconnect for business continuity and disaster recovery▶Hub and spoke LAN extension with up to 10 Gigabit Ethernet multiplexing at remote locations ▶A Flexible Mix of Advanced Optical TechnologiesNETWORK LIFE CYCLE SERVICES Fujitsu offers a broad selection of professional services to assist at every stage in a network’s evolution and operation. From planning through deployment and ongoing maintenance to future enhancements, Fujitsu Network Life Cycle Services are available whenever needed. Our comprehensive range of services includes network and system design, training, customized deployment, craft interface software, migration planning and more. Your Fujitsu sales representative can guide you in selecting the right service options for your business.Popular planning and deployment services for the FLASHWAVE 7120 managed wavelength platform include:▶ – Comprehensive verification and analysis of your installed fiber to improveF iber Characterization and Testcurrent network performance, prepare you for new growth and identify potential faults and issues.D esign Services for DWDM Networks▶ – Our professional design staff works with you to prepare a complete, custom roadmap for success.▶Turn-Up and Test – We provide a range of options from on-site configuration and simple turn-up to rigorous, long-term testing.Service Support Packages for Ongoing MaintenanceIf you’re looking for a complete professional maintenance solution, Fujitsu service support packages have the right combination of flexibility and comprehensive assurance. Choose the level and types of service you need to supplement your own resources. Our service support packages keep your network running smoothly, provide critical care and protect the longevity of your investment.Network Operations CenterWith a full range of vendor-independent network fault and performance monitoring features, the FujitsuNetwork Operations Center (NOC) offers guaranteed, round-the-clock system protection. Our reliable NOC facility is available as a primary or supplemental operations resource. This service not only helps you control costs and maintain high levels of customer satisfaction, it also provides trustworthy, reliable after-hours and emergency coverage.FEA TURES AND SPECIFICAArchitecturesPoint-to-Point (Terminal)▶Linear Optical Add/Drop▶Ring▶Maximum Number of Plug-In ModulesFLASHWAVE 7120 Shelf 23* (Passive or Active)▶F LASHWAVE 7120 Passive Shelf 2 (Passive only)▶* Note: Up to 4 shelves as a single TIDOperationsTL1 over Telnet and RS-232▶SNMP traps▶NETSMART 712 craft interface support▶NETSMART 500 craft interface support (smart launch)▶NETSMART 1500 EMS support (smart launch)▶Interoperable with Fujitsu transmission products▶Software download and remote memory backup/restore ▶Telcordia▶™ OSMINE compliantNEBS Level 3 compliant▶Optical ComponentsSingle-Channel/Sub-Band AmplificationSingle-channel/Sub-Band Booster Amplifier (SBA)▶Single-channel/Sub-Band Pre-Amplifier (SPA)▶DWDM AmplificationOptical Booster Amplifier (OBA)▶Optical Line Amplifier with Mid-stage access (OLAM)▶Optical Pre-Amplifier (OPA)▶Signal ConditioningDCM 30, 40, 60, 80 km▶LC, SC connectors Noise Filter▶Transponders/MuxpondersDual-density, multiprotocol 2.5G transponder▶Dual-density, multiprotocol 10G OTN transponder▶2:1 GigE muxponder▶4:1 multiprotocol 2.5G OTN muxponder▶10:1 multiprotocol 10G OTN muxponder▶12/24-port packet switching interfaces▶WDM FiltersCWDM 4-channel filters▶(4/8/12/16 channels) DWDM 8-channel filters▶(8/16/24/32 channels) CWDM OADM 1 channel▶DWDM OADM 1, 2 and 4 channels▶CWDM + DWDM Splitter/combiner▶Management and MonitoringIntegrated OSC/shelf controller (SCP)▶In-band OTN GCC▶Power monitoring options▶Operating EnvironmentTemperature –20 to +65 °C (–4 to +149 °F)▶Humidity 5 to 90% (non-condensing)▶Physical CharacteristicsFLASHWAVE 7120 ShelfDimensions (H x W x D) 3.5 x 17.5 x 11"▶(89 x 444 x 278 mm)Weight (fully loaded) 16.5 lb (7.5 kg)▶Power input Dual –48 V DC▶Power consumption 30 W▶Heat dissipation 102 BTU/hr▶Mounting options 19 or 23" rack mount▶Slots 6 slots▶FLASHWAVE 7120 Passive ShelfDimensions (H x W x D) 1.73 x 17.3 x 11"▶(44 x 440 x 278 mm)Weight (fully loaded) 9.1 lb (4.1 kg)▶Power input None▶Power consumption 0 W▶Heat dissipation 0 BTU/hr▶Mounting options 19 or 23" rack mount▶FLASHWAVE 7120 ShelfFujitsu Network Communications Inc.2801 Telecom Parkway, Richardson, TX 75082Tel: 800.777.FAST (3278) Fax: 972.479.6900/telecom© Copyright 2008 Fujitsu Network Communications Inc.FLASHWAVE® and NETSMART® are trademarks of Fujitsu Network Communications Inc. (USA). FUJITSU (and design)® and THE POSSIBILITIES ARE INFINITE™ are trademarks of Fujitsu Limited. All Rights Reserved.。
基于边缘检测的抗遮挡相关滤波跟踪算法

基于边缘检测的抗遮挡相关滤波跟踪算法唐艺北方工业大学 北京 100144摘要:无人机跟踪目标因其便利性得到越来越多的关注。
基于相关滤波算法利用边缘检测优化样本质量,并在边缘检测打分环节加入平滑约束项,增加了候选框包含目标的准确度,达到降低计算复杂度、提高跟踪鲁棒性的效果。
利用自适应多特征融合增强特征表达能力,提高目标跟踪精准度。
引入遮挡判断机制和自适应更新学习率,减少遮挡对滤波模板的影响,提高目标跟踪成功率。
通过在OTB-2015和UAV123数据集上的实验进行定性定量的评估,论证了所研究算法相较于其他跟踪算法具有一定的优越性。
关键词:无人机 目标追踪 相关滤波 多特征融合 边缘检测中图分类号:TN713;TP391.41;TG441.7文献标识码:A 文章编号:1672-3791(2024)05-0057-04 The Anti-Occlusion Correlation Filtering Tracking AlgorithmBased on Edge DetectionTANG YiNorth China University of Technology, Beijing, 100144 ChinaAbstract: For its convenience, tracking targets with unmanned aerial vehicles is getting more and more attention. Based on the correlation filtering algorithm, the quality of samples is optimized by edge detection, and smoothing constraints are added to the edge detection scoring link, which increases the accuracy of targets included in candi⁃date boxes, and achieves the effects of reducing computational complexity and improving tracking robustness. Adap⁃tive multi-feature fusion is used to enhance the feature expression capability, which improves the accuracy of target tracking. The occlusion detection mechanism and the adaptive updating learning rate are introduced to reduce the impact of occlusion on filtering templates, which improves the success rate of target tracking. Qualitative evaluation and quantitative evaluation are conducted through experiments on OTB-2015 and UAV123 datasets, which dem⁃onstrates the superiority of the studied algorithm over other tracking algorithms.Key Words: Unmanned aerial vehicle; Target tracking; Correlation filtering; Multi-feature fusion; Edge detection近年来,无人机成为热点话题,具有不同用途的无人机频繁出现在大众视野。
美国西蒙在MaplTG2智能基础设施中增加了互连解决方案

迹 。新 的光纤 连接解 决方 案支持 T h u n d e r b o l t 连 通 ,长 度 包 含 1 0 m、2 0 m和 3 0 m 的规 格 , 传 输速 率高达 1 0 Gb p s 。
1 1 8 智能建筑 与城市信息
2 0 1 3 年 第1 期 总第1 9 4 期
与 交 连 配 置 相 比 ,MapI T G2铜 缆 互 连
可 凭 借 其 在 可 视 化 解 决 方 案 领 域 的 多 年 经
验 , 同 时 基 于 对 客 户 需 求 的 充 分 了 解 , 为 厦航 提供 结合 6 X 3 OV L 7 1 5 L E D 显 示 屏 和 T r a n s F o r m A 图 像 控 制 器 的 定 制 化 显 示 系 统
的 数 据 中得 以证 实 。 这 一 设 备 由 技 术 人 员
在 初 始 安 装 时 和 采 取 后 续 的 移 动 、 增 加 和 改 变 时使 用 。
蔽线 缆 ,数据 主干 采用 1 2芯 室 内多模万 兆光
缆, 内外 网物理 隔离 ; 语 音干 线采 用 3 类5 0 对、 1 0 0对 大 对 数 电 缆 。
D MT O—I I 技术和世界先进的 8 . 7 Mp a德 士 古
洁 净 煤 气 化 技 术 , 高 效 清 洁 生 产 国 内 紧 缺 的 烯 烃 产 品 , 属 于 ห้องสมุดไป่ตู้ 西 省 重 大 建 设 项 目。 该 项 目共 有 4 0 0 0个 信 息 点 , 采 用 罗 格 朗 电 子 配 线架 和 L CS 系列 6类 产 品 。
有限责任公司电子配线架建设
近 日 ,蒲 城 清 洁 能 源 化 工 有 限 责 任 公 司 布 线 系统 选 用 了 T CL 一 罗 格 朗 公 司 的 综 合 布 线 解 决 方 案 。蒲城 清洁 能 源 采 用 陕西 煤业 化 工 集 团 有 限 责 任 公 司 拥 有 自 主 知 识 产 权 的
阿尔卡特朗讯助力新世界电讯在中国香港部署超高速光纤网络
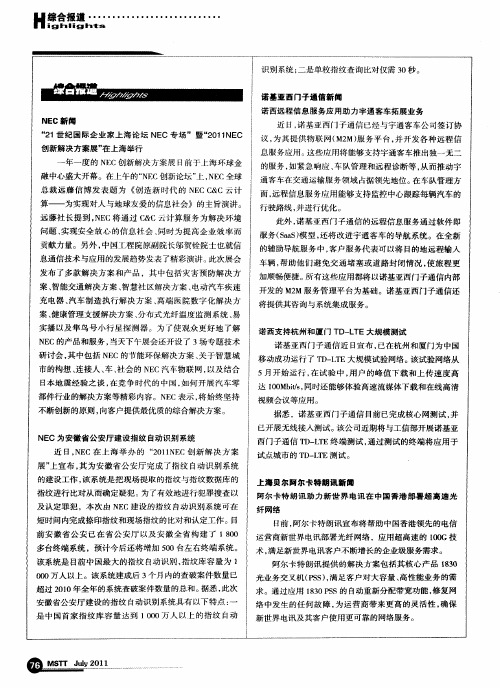
基 础 设 施 , 由 O ag uies e i s 期 与 国际 航 空 电 rne s s Sr c 近 B n ve
诺 西远 程 信 息 服 务应 用助 力字 通 客 车 拓 展业 务 近日 诺 基亚 西门子通 信已经与宇通 客车公司签订协 议 为其提供 物联 网( 2 服务平 台, M M) 并开发各种远 程信
, ,
创 新 解 决方 案展 ” 上 海举 行 在
一
年一 度 的 N C创 新 解 决 方 案 展 日前 于 上 海 环 球 金 E
。
,
是 中国首 家指纹 库容量 达到 1 0 人以 上的指 纹 自动 0万 0
新世界电讯及其客户使用更可靠 的网络服务。
…
…
…
…
…
Hale Waihona Puke …………
M
胤G 0UT D眦c  ̄ Y MI o Mc ^
通过部署支持 10 0 G传输速率 的 13 S ,新世界 电 80 S P
括从整合服 务到实时服 务管理 的端 到端 支持 。
通客车在交通运输服务领域占据领先地位 在 车 队 管 理方 面 远程信息服务应 用能够支持监控中心跟踪每辆汽车 的
。
,
远藤社 长提到 , E N C将通过 C C云计算 服务为解 决环境 &
问题 、 实现安全放 心的信息社会 、 同时为 提高企业效 率而 贡献力量 。另外 , 中国工程院原副院长邬贺铨 院士也 就信 息通信技术与应用的发展趋势发表 了精彩演讲 。 此次展会 发 布了多款解决方 案和产品 ,其 中包括灾害预 防解决方 案 、 能交通解决方案 、 智 智慧社区解 决方 案 、 电动汽车疾速 充 电器 、 汽车制造 执行解决方案 、 高端 医院数字化解 决方 案、 健康管理支援解决方案 、 布式光纤温度监测系统 、 分 易 实播以及隼 鸟号 小行 星探 测器。为 了使观众 更好地 了解 N C的产品和服务 , E 当天下午展会还开设 了 3 场专题技术
AUO Product Roadmap_20120208_Full version
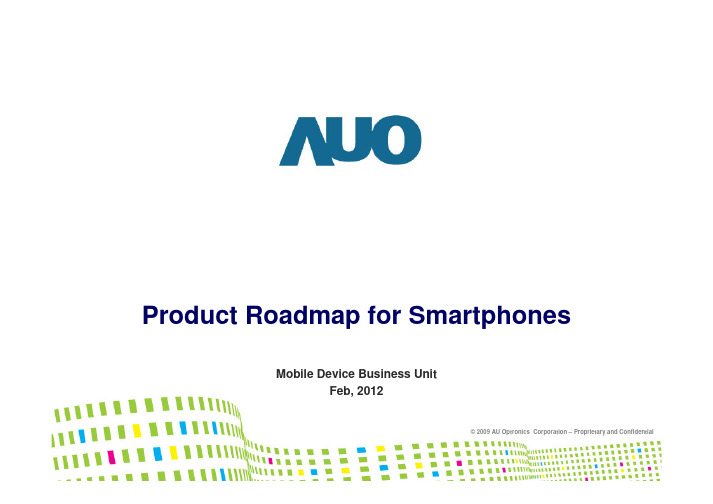
Product Roadmap for SmartphonesMobile Device Business Unit Feb, 2012© 2009 AU Optronics Corporation – Proprietary and Confidential© 2009 AU Optronics Corporation – Proprietary and ConfidentialOpen Market RoadmapMass Market Main Stream High-End3.0” H300QN 3.0” H300QN 3.2” H320QN 3.2” H320QN320x480 320x4804.02” H402VVN 4.02” H402VVN480x854 480x854 NTSC 70% NTSC 70%ES:Q2.12’ ES:Q2.12’ MP:Q2.12’ MP:Q2.12’4.29” H429AN 4.29” H429AN540x960 540x960 11’ High PPI 257 ES:Q4. 11’ High PPI 257MP:Q1.12’ MP:Q1.12’4.46” H446TAN 4.46” H446TAN1280x720 1280x720 High PPI 330 ES:Q4.11’ High PPI 330 ES:Q4.11’MP:Q2.12’ MP:Q2.12’3.17” H317QN 3.17” H317QN320x480 320x4805.0” H500VVN 5.0” H500VVN480x800 480x800 NTSC 70% NTSC 70%ES:Q1.12’ ES:Q1.12’ MP:Q2.12’ MP:Q2.12’4.46” H446AAN 4.46” H446AAN540x960 540x960 High PPI 247 High PPI 247ES:Q2.12’ ES:Q2.12’ MP:Q3.12’ MP:Q3.12’2.6” H260VVN 2.6” H260VVN (Landscape) (Landscape)480x320 480x3204.3” H430VW 4.3” H430VW480X800 480X800 NTSC 85% NTSC 85%5.3” H530AVN 5.3” H530AVN540x960 540x960 NTSC 70% NTSC 70%ES:Q2.12’ ES:Q2.12’ MP:Q3.12’ MP:Q3.12’4.3” H430VVN 4.3” H430VVN480X800 480X800 NTSC 70% NTSC 70%480X800 480X800 Slim Border 1.3mm Slim Border 1.3mm Current MP model 480X800 480X800 Slim Border 1.3mm Slim Border 1.3mm24.08” H408VW 4.08” H408VW3.8” H380VW 3.8” H380VWDeveloping model Planning model© 2009 AU Optronics Corporation – Proprietary and ConfidentialqHD (540x960) for High-end MarketKey Specification Table (qHD) Key Specification Table (qHD)H429AAN01 Model Name PPI Display Mode NTSC Contrast Ratio 257 AMVA2 70(Typ) 800(Typ) MDDI MIPI RGB 0.2t 1.7mm NT35516 Novatek 247 AHVA 70(Typ) 900(Typ) MDDI MIPI RGB 0.2 1.5mm OTM9608A Orise 208 AMVA 70(Typ) 800(Typ) MDDI MIPI RGB 0.2 1.5mm NT35516 Novatek3Key Specification Table (HD720) Key Specification Table (HD720)Model Name PPI Display Mode NTSC Contrast Ratio H446TL01 330 AHVA 70(Typ) 800(Typ)H446AAN01H530AVN01InterfaceInterfaceMDDI MIPICell Thickness Cell BorderCell Thickness Cell Border0.2t 1.6mm OTM1281A OriseCompatible ICCompatible IC© 2009 AU Optronics Corporation – Proprietary and ConfidentialWVGA (480x800) for Main-stream MarketKey Specification Table (WVGA) Key Specification Table (WVGA)Model Name H380VVN0 1 246 AMVA 70(Typ) 600(Typ) MDDI MIPI RGB 0.25t 1.3mm NT35510(N ovatek) RM68120( Raydium) H408VW01 H430VW01 H430VVN01 H430VVN01 H500VVN01PPI Display Mode NTSC Contrast Ratio Interface Cell Thickness Cell Border229 AMVA 70(Typ) 600(Typ) MDDI MIPI RGB 0.25t 1.3mm NT35510(N ovatek) RM68120(R aydium)217 AMVA 85(Typ) 800(Typ) MDDI MIPI RGB 0.3t 2.0mm NT35510(N ovatek) RM68120(R aydium)4217 AMVA 70(Typ) 800(Typ) MDDI MIPI RGB 0.3t 1.7mm NT35510(N ovatek) RM68120(R aydium)217 AMVA 70(Typ) 800(Typ) MDDI MIPI RGB 0.3t 1.7mm NT35510(N ovatek) RM68120(R aydium)186 AMVA 70(Typ) 800 (Typ) MDDI MIPI RGB 0.3t 2.0mm RM68120(R aydium)Compatible IC© 2009 AU Optronics Corporation – Proprietary and ConfidentialDisplay Size / Resolution Migration for SmartphonesFWVGA FWVGAModel Name PPI Display Mode NTSC Contrast Ratio Interface Cell Thickness Cell Border Compatible ICH402VAN01Key Specification Table (HVGA) Key Specification Table (HVGA)Model Name H300QN01 H317QN01 H320QN01 H260VVN01 (Landscape) 222 AMVA 70(Typ) 800(Typ)246PPI 192 AMVA 50(Typ) 500(Typ) 182 AMVA 60(Typ) 300(Typ) 180 AMVA 70(Typ) 500(Typ)AMVA 70(Typ)Display Mode NTSC800(Typ) MDDI MIPI RGB 0.25t 1.5mm NT35510 OTM8009AContrast RatioInterfaceMDDI / MIPI RGB CPUMDDI / MIPI RGB CPUMDDI / MIPI RGB CPUMDDI RGB CPUCell Thickness Compatible IC0.25t0.3t0.2t0.2t R61531 RSP---© 2009 AU Optronics Corporation – Proprietary and Confidential54.08” & 3.97” WVGA Performance Comparison4.08” WVGA 3.97” WVGA51.84x86.4Remark 螢幕較大貼近市場趨勢4” 以上 以上Smartphone手握寬幅約在 手握寬幅約在 64~65mm左右,AUO Slim Border可 左右, 可 左右 縮小差異僅到左右0.22mm,藉由機構 縮小差異僅到左右 , Cover微小差異。
可重构智能超表面在卫星导航系统中的应用展望
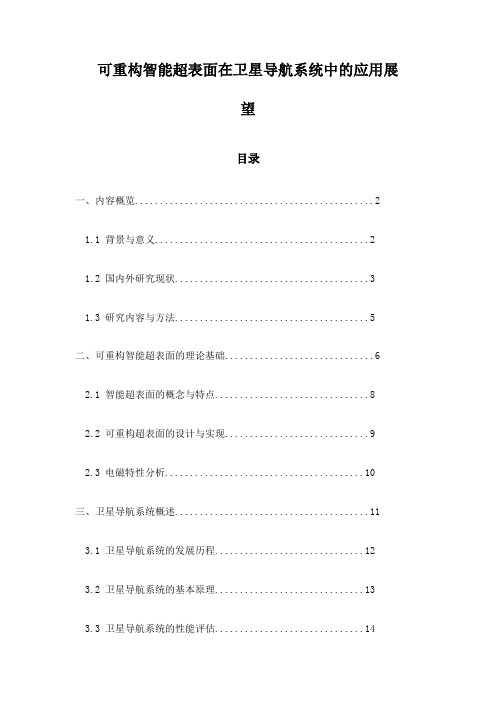
可重构智能超表面在卫星导航系统中的应用展望目录一、内容概览 (2)1.1 背景与意义 (2)1.2 国内外研究现状 (3)1.3 研究内容与方法 (5)二、可重构智能超表面的理论基础 (6)2.1 智能超表面的概念与特点 (8)2.2 可重构超表面的设计与实现 (9)2.3 电磁特性分析 (10)三、卫星导航系统概述 (11)3.1 卫星导航系统的发展历程 (12)3.2 卫星导航系统的基本原理 (13)3.3 卫星导航系统的性能评估 (14)四、可重构智能超表面在卫星导航系统中的应用原理 (15)4.1 基于可重构智能超表面的波束赋形 (17)4.2 基于可重构智能超表面的信号处理 (18)4.3 基于可重构智能超表面的抗干扰能力 (20)五、可重构智能超表面在卫星导航系统中的性能优化 (21)5.1 性能评估指标体系建立 (22)5.2 性能优化方法探讨 (24)5.3 实验验证与分析 (26)六、可重构智能超表面在卫星导航系统中的实际应用场景 (27)6.1 智能交通系统中的应用 (28)6.2 物联网中的定位与导航 (30)6.3 军事通信系统中的应用 (31)七、结论与展望 (32)7.1 研究成果总结 (33)7.2 存在的问题与挑战 (34)7.3 未来发展方向与前景展望 (35)一、内容概览随着科技的飞速发展,智能化、高精度导航系统已成为现代社会的迫切需求。
作为导航系统的核心组件,地面和卫星导航信号处理单元在定位、导航和授时等方面发挥着至关重要的作用。
传统的地面和卫星导航信号处理单元在面对复杂多变的环境时,往往显得力不从心,难以满足日益增长的导航精度和可靠性要求。
在此背景下,可重构智能超表面作为一种新兴的技术手段,为卫星导航系统的发展带来了新的机遇和挑战。
可重构智能超表面是一种通过改变其物理结构来实现功能调整的超表面,具有灵活性高、适应性强等优点。
将其应用于卫星导航系统,可以有效地提高信号处理能力,增强抗干扰性能,从而提升整个导航系统的稳定性和准确性。
超高速超长距光波分复用系统通道代价计算模型研究
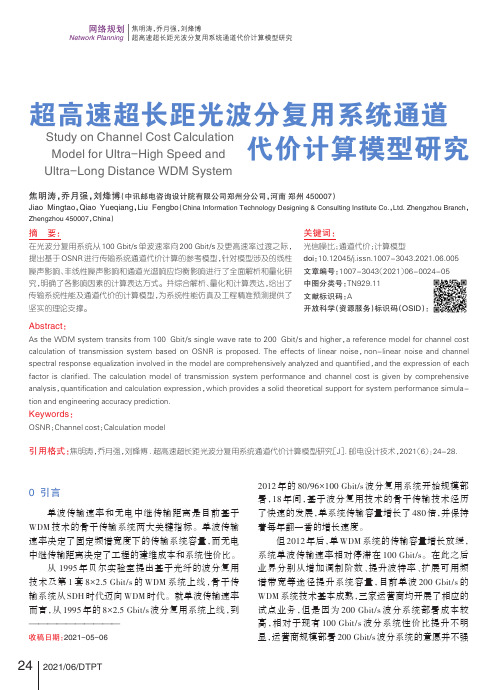
究,明确了各影响因素的计算表达方式。并综合解析、量化和计算表达,给出了 中图分类号:TN929.11
传输系统性能及通道代价的计算模型,为系统性能仿真及工程精准预测提供了 文献标识码:A
坚实的理论支撑。
开放科学(资源服务)标识码(OSID):
Abstract:
As the WDM system transits from 100 Gbit/s single wave rate to 200 Gbit/s and higher,a reference model for channel cost calculation of transmission system based on OSNR is proposed. The effects of linear noise,non-linear noise and channel spectral response equalization involved in the model are comprehensively analyzed and quantified,and the expression of each factor is clarified. The calculation model of transmission system performance and channel cost is given by comprehensive analysis,quantification and calculation expression,which provides a solid theoretical support for system performance simulation and engineering accuracy prediction.
- 1、下载文档前请自行甄别文档内容的完整性,平台不提供额外的编辑、内容补充、找答案等附加服务。
- 2、"仅部分预览"的文档,不可在线预览部分如存在完整性等问题,可反馈申请退款(可完整预览的文档不适用该条件!)。
- 3、如文档侵犯您的权益,请联系客服反馈,我们会尽快为您处理(人工客服工作时间:9:00-18:30)。
On-Chip Optical Interconnect Roadmap:Challengesand Critical DirectionsMikhail Haurylau,Associate Member,IEEE,Guoqing Chen,Hui Chen,Jidong Zhang,Nicholas A.Nelson,Student Member,IEEE,David H.Albonesi,Senior Member,IEEE,Eby G.Friedman,Fellow,IEEE,and Philippe M.Fauchet,Fellow,IEEEAbstract—Intrachip optical interconnects(OIs)have the poten-tial to outperform electrical wires and to ultimately solve the com-munication bottleneck in high-performance integrated circuits. Performance targets and critical directions for ICs progress are yet to be fully explored.In this paper,the International Technol-ogy Roadmap for Semiconductors(ITRS)is used as a reference to explore the requirements that silicon-based ICs must satisfy to successfully outperform copper electrical interconnects(IEs). Considering the state-of-the-art devices,these requirements are extended to specific IC components.Index Terms—Integrated optoelectronic circuits,optoelectron-ics,optical interconnects(ICs),silicon photonics.I.I NTRODUCTIONT HE communications bottleneck is identified as one of the grand challenges in the progress of silicon computa-tion.1While individual logic elements have become significantly faster,computational speed is limited by the communication be-tween different parts of a processor.Optical interconnects(OIs) can provide a solution to the communication bottleneck by re-placing electrical wires with faster optical waveguides[1]. Three levels of interconnects can be identified:1)board-to-board;2)chip-to-chip;and3)intrachip.While OIs on the backplane and interchip levels are actively under development now[2],whether intrachip OIs are feasible remains an open question.To support the sufficient density of interconnections and integration with CMOS processing,OIs should be mono-lithically fabricated using CMOS compatible silicon-based ma-terials and processes.Until recently,such devices did not exist. Over the past few years,significant progress has been made in the development of silicon-based building blocks for on-chip OIs,including light sources[3],[4],[5],waveguides[6],mod-ulators[7],[8],and detectors[9],[10].While some predictions have been made[11],[12],there is as yet no clear performance specifications for intrachip optical components to effectively replace electrical interconnects(EIs)[13].In this paper,the International Technology Roadmap for Semiconductors(ITRS)is used to predict the EI performance, Manuscript received December24,2005;revised May30,2006.This work was supported by the National Science Foundation under Grant CCR-0304574.M.Haurylau,G.Chen,H.Chen,J.Zhang,N.A.Nelson,E.G.Fried-man,and P.M.Fauchet are with the Department of Electrical and Computer Engineering,University of Rochester,Rochester,NY14627USA(e-mail: haurylau@).D.H.Albonesi is with the Department of Electrical and Computer Engineer-ing,Cornell University,Ithaca,NY14853USA.Digital Object Identifier10.1109/JSTQE.2006.8806151[Online].Available:/as a target for OI requirements.1From an analysis of parameters such as delay,bandwidth density,and power consumption,the requirements for individual OI components are identified.While the delay is an important metric for interconnect performance, the power and area budgets are as important for determining system performance.Our paper also identifies OI weaknesses and missing components.Finally,it is important to differentiate between local and global intrachip interconnects.Local interconnects have a delay of less than one clock cycle,while global interconnects typically take longer than one or two clock cycles.Local interconnects are used for short-distance communication and comprise the major-ity of on-chip wires.While there are fewer global interconnects, these links are no less important.Improving the performance of a small number of critical global links can significantly en-hance the total system performance.Section II shows that OIs are better suited for long-distance communications.Therefore,a comparison between electrical and optical global interconnects is the primary focus of this paper.II.EIs R OADMAPModern on-chip EIs utilize copper wires surrounded by a low-k dielectric to transmit a signal[14].Long wires used for global interconnects tend to exhibit higher RC time constants,which increases the interconnect delay,transition time,and crosstalk noise.In submicrometer CMOS technologies,repeaters[15] (or electrical signal amplifiers)are widely used to break long wires into smaller parts,as shown in Fig.1(a).Repeaters drive smaller individual wire segments,thereby reducing the overall interconnect delay and making the overall delay linear with line length rather than quadratic.The delay due to the wires becomes smaller when the number of repeaters increases,but there is a delay and power penalty associated with the repeater circuitry.Therefore,for afixed interconnect geometry,there exists an optimal repeater size and spacing to achieve a minimum delay[16].In the following discussion,only EIs with optimized repeaters are considered.When modeling metal wire interconnects operating at multi-gigahertz clock rates,it is important to consider three impedance characteristics of the wire—resistance,capacitance,and induc-tance.In this paper,an RLC interconnect with equally spaced repeaters is examined for different technology nodes.1Three degrees of freedom—the wire width,and the number and size of the repeaters—are explored to determine the minimum signal propagation delay.The delay model for the interconnect is an1077-260X/$20.00©2006IEEEFig.1.(a)Circuit schematic of the EI system.(b)Block diagram of the OIsystem.Fig.2.Propagation delay of EI at different technology nodes versus normal-ized interconnect width.extension of work described in [17]and includes the effects of repeater output capacitance and input signal transition time.Two of the main parameters characterizing on-chip intercon-nects are the propagation delay and the interconnect bandwidth density.The EI delay can be reduced by increasing the inter-connect width at the expense of a smaller bandwidth density.In Fig.2,the minimum EI delay per unit length is plotted as a function of wire width for different technology nodes.Note that technology scaling has insignificant effect on the delay of an interconnect with an optimal number of repeaters.The mini-mum achievable interconnect delay remains effectively fixed at approximately 20ps/mm when technology scales from 90nm (year 2004)to 22nm (year 2016).Here,the maximum bit rate for a single interconnect is assumed to be the clock rate.With this assumption,the bandwidth density increases due to the smaller wire pitch and higher clock rate.There are two major strategies for designing interconnects.Bandwidth density optimized interconnects utilize minimum-sized wires but exhibit a large RC impedance.Delay optimized interconnects sacrifice bandwidth density in favor of lower de-lay by using wider wires.OIs are likely to initially benefit globalinterconnections,as EI-based global interconnects are typically delay-limited.Therefore,only delay-optimized EIs are consid-ered for comparison with OIs.The estimated power consump-tion per unit length for delay-optimized EIs with optimal re-peaters is of the order of 1mW/mm and is expected to slowly increase [12].From this analysis,technology scaling is not expected to significantly change the EI delay;however,the EI bandwidth density is expected to increase with time.Therefore,progress in OIs must recognize that the performance of intrachip EIs is a moving (and improving)target.III.OIs:C ONFIGURATION AND A DV ANTAGESA.Monolithic VLSI Technology:Advantages and LimitationsThe introduction of OIs into high-performance,high-complexity integrated circuits requires monolithic integration with standard electronic logic circuits.Microelectronic mono-lithic fabrication is perhaps one of the most robust and high-yield technologies in modern industry,resulting in low cost and ul-trahigh levels of device integration.The number of materials and processes available for OI fabrication,however,is limited to those technologies that are compatible with microelectronics.An important consequence of these limitations is the absence of efficient monolithic on-chip light sources.While a number of exciting scientific achievements have recently been published in the area of optical gain in silicon [3],[18],[19],high-speed,electrically driven,monolithic light sources are far from reality.It is,therefore,assumed that the most likely optical transmitter configuration is a silicon-compatible electro-optic modulator with an external laser light source.In this paper,an OI system that consists of three main parts is considered:1)an on-chip light modulator for signal switching;2)a waveguide to guide the light;and 3)a photodetector as a receiver.This system is illustrated in Fig.1(b).An off-chip laser is assumed to be the light source for the OI system.The modulator and detector have conflicting requirements with respect to light absorption.The modulator material should be transparent to minimize insertion losses,while the detector material must absorb light to generate charge carriers.Thus,different materials should be used as detector and modulator,e.g.,germanium and silicon.As a result,the wavelength range between the absorption edges in silicon and germanium defines the available wavelengths for signal modulation and detection.B.Optical Waveguides:Delay AdvantageMinimizing the signal propagation delay is the primary in-terconnect requirement for the majority of very large scale in-tegration (VLSI)architectures.In this respect,OIs possess the intrinsic advantage of high signal propagation speed in optical waveguides,especially when the signal dispersion is negligible.A comparison of signal propagation delay in EI and the two common types of optical waveguides—a polymer waveguide and a silicon waveguide—is shown in Fig.3.Low refractive index polymer and high refractive index silicon waveguides are chosen for comparison with EIs,as these structures representHAURYLAU et al.:ON-CHIP OPTICAL INTERCONNECT ROADMAP:CHALLENGES AND CRITICAL DIRECTIONS1701Fig.3.Propagation delay of silicon and polymer waveguides as compared to EIs.The R-Soft full-vectorialfinite-difference time-domain(FDTD)solver has been used to determine the waveguide properties.Both types of optical waveguides have a square cross section and are assumed to be surrounded by a cladding with a refractive index of1.1.The core of the silicon waveguide is0.34µm wide and has a refractive index of3.4.The core of the polymer waveguide is1.36µm wide and has a refractive index of1.3.two opposite types of optical waveguides in terms of signal propagation delay and crosstalk.Note that optical waveguides provide a significant advantage in propagation delay over elec-trical wires regardless of the waveguide material.Optical signal propagation is intrinsically faster than electrical propagation due to the absence of RLC impedances.While the majority of VLSI architectures are delay-limited,an effective choice for intrachip interconnects are low-delay polymer waveguides that can be realized,e.g.,with low-loss optical polymers[20].In order to exploit the propagation delay advantage offered by optical waveguides,it is necessary tofirst convert the elec-trical signal into light and then back into an electrical signal. This conversion has afixed delay,which is nearly independent of the interconnect length for a given technology.Hence,OIs tend to have a delay advantage in longer connections,when the waveguide propagation delay dominates the overall delay.IV.OIs V ERSUS EIsA.Transmitter and Receiver:Conversion Costand Power-Delay ProductTo be considered as a candidate for replacing EIs,OIs should exhibit advantages in both delay and power for critical long-distance intrachip interconnections.If the average length of the global interconnects in a target architecture is known,it is possi-ble to extract the conversion cost(i.e.,delay and power)require-ment for OIs.As an example,the OI conversion requirements for an interconnect length equal to the ITRS projected chip edge length of17.6mm for both polymer-core and silicon-core waveguides are shown in Fig.4.In thisfigure,the EI delay is plotted as a function of distance.The optical waveguide delay is then projected back from the17.6-mm EI delay to the y-axis as indicated by the arrow.The y-intercept of the optical waveguide delay curve indicates the maximum allowed conversion delayτin the chip edge length OI.As illustrated in Fig.4,the combined transmitter and receiver delay should be lower than280–370ps for polymer waveguides and180–270ps for siliconwaveguides.Fig.4.Maximum conversion cost for an on-chip OI.Left axis shows the signal propagation delay and right axis the power consumption in the interconnect.τM P is the maximum allowed electrical–optical–electrical conversion delay in the chip edge length polymer waveguide OI,τM Si is the maximum allowed conversion delay in the chip edge length silicon waveguide OI,and P M is the maximum allowed conversion power consumption in the chip edge lengthOI.Fig.5.Circuit schematic of(a)waveguide receiver and(b)modulator driver. Using the second y-axis in a similar way,the total power con-sumption should be less than17–18mW for chip-length OI. The conversion penalty consists of two parts—the transmitter and receiver.The receiver consists of a photodetector that con-verts light into electricity and receiver circuitry that amplifies and converts the analog electrical signal into a digital voltage signal[see Fig.5(a)].The key issue to be addressed in the design of a photodetector is the tradeoff between detector speed and quantum efficiency(or sensitivity of the detector).Interdigitated metal–semiconductor–metal(MSM)receivers have attracted at-tention due to the fast response and excellent quantum efficiency. Recently,there have been a number of reports on high-speed, low-power interdigitated MSM Ge and SiGe photodetectors op-erating at telecommunication wavelengths[9],[10].As com-pared to other Si-based photonic components,the reported per-formances already satisfy the requirements of on-chip global1702IEEE JOURNAL OF SELECTED TOPICS IN QUANTUM ELECTRONICS,VOL.12,NO.6,NOVEMBER/DECEMBER2006bined power-delay product for interdigitated MSM photodetector and receiver circuits.interconnect.By optimizing the interdigitated electrode width, the detector bandwidth can be further increased.The power and delay product(PDP)is routinely used in the technology design process to evaluate circuit performance.Sim-ulations of the PDP of a Ge MSM detector and receiver circuits are illustrated in Fig.6.Note that there exists an electrode spac-ing at which the PDP is lowest.This minimum is a compromise between the longer carrier transit time for larger electrode spac-ings and the increase in the RC impedance for small electrode spacings[21].Also note that the optimum electrode spacing differs,depending upon the detector size.Both the delay and power consumption can be further reduced by decreasing the size of the detector.The current state of electro-optic transmitters suitable for intrachip OIs is much less advanced.A transmitter consists of a modulator and driver circuits[see Fig.5(b)].A series of tapered inverters is used to drive the modulator[12].Although significant progress has recently been made[7],[8]in silicon-based modulators,these modulators do not currently provide the necessary performance to replace EIs.The main parameter for a modulator is an effective refractive index change∆n effin the active area,or the phase shifter of the modulator.The higher∆n eff,the more compact the modulator,thereby reducing the propagation delay and power consumption.The two main types of modulators are Mach–Zehnder interferometer-based modulators[7]and microresonator-based modulators[8](see Fig.7).For modulators with a Mach–Zehnder interferometer structure,∆n effdetermines the length of the phase shifters.For microresonator-based modulators,∆n effdetermines the value of the resonance wavelength shift and therefore the extinction ratio,or the on/off contrast.The dependence of the PDP of the modulator and driver cir-cuits on∆n efffor both Mach–Zehnder interferometers-and microresonator-based modulators with driver circuits is shown in Fig.8.The modulator load is modeled as a simple capacitor and is assumed to scale linearly with the modulator length at the rate of1.7pF/mm.Both the length and delay of a Mach–Zehnder modulator are determined by∆n eff.The length of the active re-gion of a microresonator-based modulator is assumed to be con-stant;therefore,∆n effonly affects the delay.The structureof Fig.7.Schematic of a Mach–Zehnder modulator.(a)Interferometer based.(b)Microresonatorbased.bined power-delay product of transmitter as a function of∆n efffor the90nm technology node.Delay and power consumption of both the modu-lator and receiver circuits are included in the PDP.A series of optimized tapered inverters[15]is used to drive the modulator.The transimpedance amplifier is used to amplify the photocurrent from the detector.Additional minimum-sized inverters are used to amplify the signal to a digital level.For comparison,the dashed line describes the PDP of a10-mm-long EI.a microresonator-based modulator is a generalized Fabry–Perot cavity,with the dimensions of the phase shifter limited by the cavity size.The PDP model is valid for any resonant structure, including two-dimensional(2-D)photonic bandgap microcavi-ties and microring resonators as long as the active region is no larger than the cavity size.The PDP of a delay-optimized EI for a90nm technology node is also shown for comparison.Fig.8shows that the PDP of a microresonator-based mod-ulator is significantly better than that of a Mach–Zehnder in-terferometer.Microresonator-based modulators can effectively fold the active device region,thereby significantly reducing the power consumption and the driver delay.Resonant structures should easily exceed EIs in terms of the PDP,as shown in Fig.8.While microresonators are superior to Mach–Zehnder interferometers,there are two problems that should be solved before resonant-based structures can be successfully used for intrachip applications.First,microresonators have a low fabri-cation tolerance.This factor may become less important as litho-graphic techniques improve.Second,unlike Mach–Zehnder in-terferometers,microresonators are susceptible to temperature fluctuations due to the d n/d T of the cavity material.While the introduction of OIs may help manage the thermal budgetHAURYLAU et al.:ON-CHIP OPTICAL INTERCONNECT ROADMAP:CHALLENGES AND CRITICAL DIRECTIONS1703in multi-core processor architectures[13],OIs remain suscepti-ble to temperature variations.Either an active or passive optical control method similar to that published in[22]is required to maintain stable device operation.B.Bandwidth Density ComparisonBandwidth density is a metric that characterizes information throughput through a unit cross section of an interconnect.Gen-erally,it is defined by the pitch of the electrical wires in EIs or optical waveguides in OIs.Optical waveguides can be reliably compared to EIs,since the size and propagation delay can be straightforwardly determined.Each type of optical waveguide produces a different propagation delay and bandwidth density, both of which are determined by the waveguide geometry and the index contrast between the waveguide core and cladding.The minimum pitch between two adjacent waveguides is determined by the crosstalk considerations.For a particular waveguide ma-terial,an optimum ratio exists between the waveguide width w and pitch p.With afixed pitch,if a waveguide is too wide, the crosstalk is high due to the proximity between the sides of adjacent waveguides.If the waveguide is too narrow,the optical mode becomes less confined,causing a higher crosstalk due to a larger overlap between adjacent optical modes.This tradeoff for polymer waveguides is illustrated in Fig.9(a).To estimate the maximum bandwidth density,the minimum waveguide pitch is determined by setting the crosstalk limit to 20%in a10-mm long interconnect.The optical signal propaga-tion delay is determined from the n effof the simulated optical mode.The resulting tradeoff is depicted in Fig.9(b),where the waveguide delay and minimum pitch are plotted versus the re-fractive index of the core.A general trend is that a high-index core offers a smaller waveguide pitch,while a low-index core offers a lower propagation delay.This graph can be used to eval-uate the two essential interconnect requirements—propagation delay and bandwidth density.As illustrated in Fig.10,optical waveguides should be spaced approximately0.5–3µm from each other to avoid significant crosstalk.In contrast,a delay-optimized pitch for electrical wires is aroundfive to seven node sizes,providing a significant ad-vantage in bandwidth density.A comparison of the bandwidth density for delay-optimized EIs and optical waveguides is il-lustrated in Fig.10.The increase in optical bandwidth density shown in the graph is due solely to the higher bit rate through the waveguides with afixed pitch.EIs can also exploit more efficient repeaters,resulting in a higher growth in bandwidth density.Therefore,a single wavelength optical link is infe-rior to a delay-optimized electrical wire in terms of bandwidth density.A viable solution to the bandwidth density problem in OIs is to use wavelength division multiplexing(WDM)to enhance the OI bandwidth density.The number of WDM channels required to match the EI bandwidth density for both SOI and polymer waveguides is shown in Fig.11.Two types of tradeoffs can be identified from this graph.Polymer-core waveguides require higher WDM to match the bandwidth density but allow for a larger conversion delay overhead.Silicon-corewaveguides,Fig.9.Optical waveguide analysis.(a)Sketch of the modeled waveguides (inset)and the crosstalk as a function of the w/p ratio for a10-mm polymer-core waveguide interconnect.When w/p is too small,the crosstalk is high due to a smaller mode confinement,whereas when w/p is too large,the crosstalk is high because the waveguide walls are too close.(b)The tradeoff between waveguide density and propagation delay per unit length.The graph is plotted for a10\,mm interconnect and a maximum allowed crosstalk of20%.The height h and width w of all of the waveguides are set equal.The cladding material is assumed to have a refractive index of1.1,and the wavelength of light is1.3µm. The optimum w/p ratio for each data point is determinedseparately.parison of bandwidth density of electrical wires and OIs as a function of year and technology node.For reference,the thin solid line illustrates the ITRS prediction for the clock rate.Bandwidth density is an important metric, defining the information throughput of an interconnect through a unit cross section.1704IEEE JOURNAL OF SELECTED TOPICS IN QUANTUM ELECTRONICS,VOL.12,NO.6,NOVEMBER/DECEMBER2006Fig.11.Number of OI WDM channels required to exceed the EI bandwidth density as a function of year.however,permit lower WDM but require faster transmitters and receivers.Note that while only a moderate number of WDM channels is required to match the EI bandwidth density,there is an area and delay penalty associated with addition of each WDM channel.Resonant structures are naturally suited for WDM ar-chitectures and can help reduce the WDM overhead.V.C ONCLUSION AND U PCOMING C HALLENGES Based on the semiconductor technology roadmap,require-ments and critical directions are presented for intrachip OIs. From this discussion,the following requirements should be sat-isfied for OIs to be competitive with EIs for intrachip global interconnects.1)The combined transmitter and receiver delays should belower than280–370ps for polymer waveguides and lower than180–270ps for silicon waveguides for chip-length global interconnect.2)The total power consumption should be comparable to thatof EI(∼18mW)for chip-length global interconnect. 3)The maximum bandwidth,or bit rate,should exceed theITRS prediction for the clock rate.4)Since the bandwidth density is expected to grow for EIs,anincreasing number of WDM channels is necessary for OIs to exceed EI performance,up to nine in the case of low-index waveguides and three for high-index waveguides by the year2016.5)General CMOS requirements,most significantly technol-ogy compatibility and temperature stability,should be sat-isfied.This discussion has identified the primary challenges for in-trachip OIs to successfully compete with EIs.First,the size, delay,and power consumption of silicon-compatible modula-tors should be significantly reduced before any state-of-the-art modulator can be considered for on-chip applications.Second, the introduction of WDM requires the development of ultra-compact integrated wavelength-selective components and effi-cient broadband external lasers.Finally,passive or active tem-perature drift compensation is necessary to ensure reliable op-eration of OIs.R EFERENCES[1]J.W.Goodman,F.J.Leonberger,S.Y.Kung,and R.A.Athale,“Opticalinterconnections for VLSI systems,”Proc.IEEE,vol.72,no.7,pp.850–866,Jul.1984.[2]N.Savage,“Linking with light,”IEEE Spectr.vol.39,no.8,pp.32–36,Aug.2002.[3]H.Rong,R.Jones,A.Liu,O.Cohen,D.Hak,A.Fang,and M.Paniccia,“A continuous-wave Raman silicon laser,”Nature,vol.433,pp.725–728, Feb.2005.[4]P.M.Fauchet,“Light emission from Si quantum dots,”Mater.Today,vol.8,no.1,pp.26–31,Jan.2005.[5]L.Pavesi,“Routes toward silicon-based laser,”Mater.Today,vol.8,no.1,pp.18–25,Jan.2005.[6]S.J.McNab,N.Moll,and Yu.A.Vlasov,“Ultra-low loss photonic in-tegrated circuit with membrane-type photonic crystal waveguides,”Opt.Express,vol.11,no.22,pp.2927–2939,Nov.2003.[7] A.Liu,R.Jones,L.Liao,D.Samara-Rubio,D.Rubin,O.Cohen,R.Nico-laescu,and M.Paniccia,“A high-speed silicon optical modulator based on a metal-oxide-semiconductor capacitor,”Nature,vol.427,no.6975, pp.615–618,Feb.2004.[8]Q.F.Xu,B.Schmidt,S.Pradhan,and M.Lipson,“Micrometre-scalesilicon electro-optic modulator,”Nature,vol.435,no.7040,pp.325–327,May2005.[9]M.R.Reshotko,D.L.Kencke,and B.Block,“High-speed CMOS com-patible photodetectors for optical interconnects,”Proc.SPIE,Oct.2004, vol.5564,pp.146–155.[10]S.J.Koester,J.D.Schaub,G.Dehlinger,J.O.Chu,Q.C.Ouyang,andA.Grill,“High-ffficiency,Ge-on-SOI lateral PIN photodiodes with29GHz bandwidth,”in Proc.Device Research Conf,Notre Dame,IN,2004, pp.175–176.[11]M.J.Kobrinsky,B.A.Block,J.-F.Zheng,B.C.Barnett,E.Mohammed,M.Reshotko,F.Robertson,S.List,I.Young,and K.Cadien,“On-chip optical interconnects,”Intel Technol.J.,vol.8,no.2,pp.129–141,May 2004.[12]G.Chen,H.Chen,M.Haurylau,N.Nelson,D.H.Albonesi,P.M.Fauchet,and E.G.Friedman,“Predictions of CMOS compatible on-chip optical interconnect,”in Proc.ACM/IEEE Int.Workshop Syst.Level Interconnect Prediction,San Francisco,CA,2005,pp.13–20.[13]N.Nelson,G.Briggs,M.Haurylau,G.Chen,H.Chen,D.H.Albonesi,E.G.Friedman,and P.M.Fauchet,“Alleviating thermal constraints whilemaintaining performance via silicon-based on-chip optical interconnects,”in Proc.Workshop Unique Chips and Systems,Austin,TX,2005,pp.45–52.[14]R.Ho,K.W.Mai,and M.A.Horowitz,“The future of wires,”Proc.IEEE,vol.89,no.4,pp.490–504,Apr.2001.[15]V.Adler and E.G.Friedman,“Repeater design to reduce delay and powerin resistive interconnect,”IEEE Trans.Circuits Syst.II,Analog Digital Signal Process,vol.45,no.5,pp.607–616,May1998.[16] B.S.Cherkauer and E.G.Friedman,“A unified design methodology forCMOS tapered buffers,”IEEE Trans.Very Large Scale(VLSI)Integr.Syst.,vol.3,no.1,pp.99–111,Mar.1995.[17]Y.I.Ismail and E.G.Friedman,“Effects of inductance on the propagationdelay and repeater insertion in VLSI circuits,”IEEE Trans.Very Large Scale(VLSI)Integr.Syst.,vol.8,no.2,pp.195–206,Apr.2000.[18]J.Ruan,P.M.Fauchet,L.Dal Negro,M.Cazzanelli,and L.Pavesi,“Stimulated emission in nanocrystalline silicon superlattices,”Appl.Phys.Lett.,vol.83,no.26,pp.5479–5481,Dec.2003.[19]O.Boyraz and B.Jalali,“Demonstration of a silicon Raman laser,”Opt.Express,vol.12,no.21,pp.5269–5273,Oct.2004.[20]L.Eldada and L.W.Shacklette,“Advances in polymer integrated optics,”IEEE J.Sel.Topics Quantum Electron.,vol.6,no.1,pp.54–68,Jan.2000.[21]S.V.Averine,Y.C.Chan,and m,“Geometry optimization of inter-digitated Schottky-barrier metal–semiconductor–metal photodiode struc-tures,”Solid-State Electron.,vol.45,no.3,pp.441–446,Mar.2001. [22]S.M.Weiss,M.Molinari,and P.M.Fauchet,“Temperature stability forsilicon-based photonic band-gap structures,”Appl.Phys.Lett.,vol.83, no.10,pp.1980–1982,Sep.2003.。