Fatigue for Engineers
疲劳分析软件介绍_DesignLife_9-1_S2098-6_EN_web
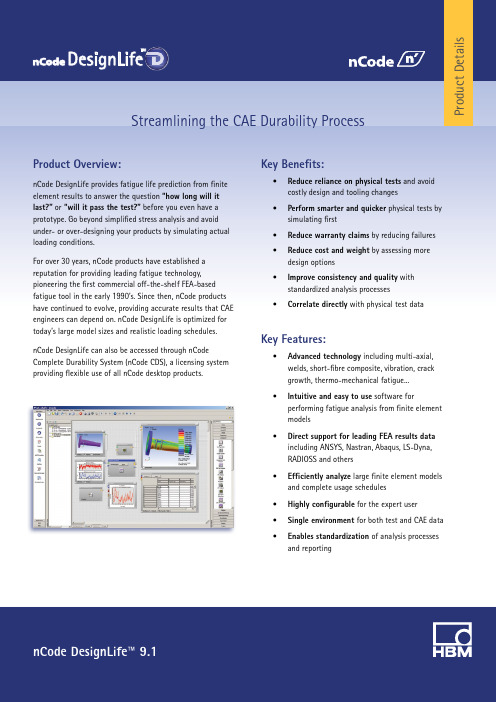
Streamlining the CAE Durability Process
Product Overview:
nCode DesignLife provides fatigue life prediction from finite element results to answer the question “how long will it last?” or “will it pass the test?” before you even have a prototype. Go beyond simplified stress analysis and avoid under- or over-designing your products by simulating actual loading conditions.
• Direct support for leading FEA results data including ANSYS, Nastran, Abaqus, LS-Dyna, RADIOSS and others
• Efficiently analyze large finite element models and complete usage schedules
• Crack Growth – provides a complete fracture mechanics capability using industry standard methodologies for specified locations on FE model. Built-in growth laws include NASGRO, Forman, Paris, Walker and more. Select from a provided library of geometries or supply custom stress intensity factors.
钢结构毕业设计论文(中英)
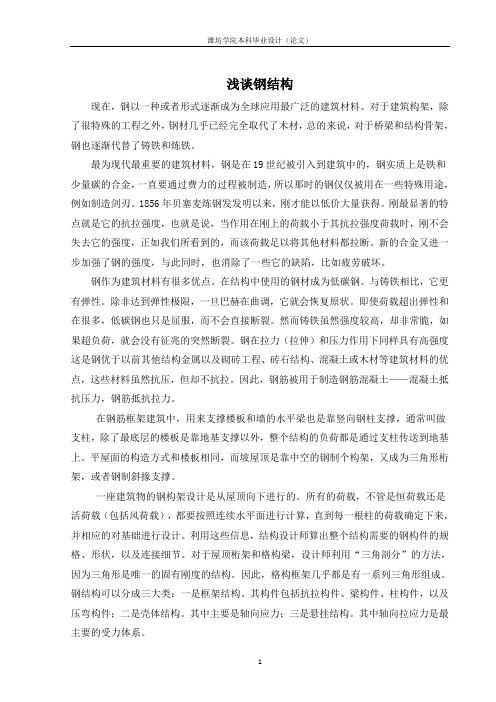
浅谈钢结构现在,钢以一种或者形式逐渐成为全球应用最广泛的建筑材料。
对于建筑构架,除了很特殊的工程之外,钢材几乎已经完全取代了木材,总的来说,对于桥梁和结构骨架,钢也逐渐代替了铸铁和炼铁。
最为现代最重要的建筑材料,钢是在19世纪被引入到建筑中的,钢实质上是铁和少量碳的合金,一直要通过费力的过程被制造,所以那时的钢仅仅被用在一些特殊用途,例如制造剑刃。
1856年贝塞麦炼钢发发明以来,刚才能以低价大量获得。
刚最显著的特点就是它的抗拉强度,也就是说,当作用在刚上的荷载小于其抗拉强度荷载时,刚不会失去它的强度,正如我们所看到的,而该荷载足以将其他材料都拉断。
新的合金又进一步加强了钢的强度,与此同时,也消除了一些它的缺陷,比如疲劳破坏。
钢作为建筑材料有很多优点。
在结构中使用的钢材成为低碳钢。
与铸铁相比,它更有弹性。
除非达到弹性极限,一旦巴赫在曲调,它就会恢复原状。
即使荷载超出弹性和在很多,低碳钢也只是屈服,而不会直接断裂。
然而铸铁虽然强度较高,却非常脆,如果超负荷,就会没有征兆的突然断裂。
钢在拉力(拉伸)和压力作用下同样具有高强度这是钢优于以前其他结构金属以及砌砖工程、砖石结构、混凝土或木材等建筑材料的优点,这些材料虽然抗压,但却不抗拉。
因此,钢筋被用于制造钢筋混凝土——混凝土抵抗压力,钢筋抵抗拉力。
在钢筋框架建筑中,用来支撑楼板和墙的水平梁也是靠竖向钢柱支撑,通常叫做支柱,除了最底层的楼板是靠地基支撑以外,整个结构的负荷都是通过支柱传送到地基上。
平屋面的构造方式和楼板相同,而坡屋顶是靠中空的钢制个构架,又成为三角形桁架,或者钢制斜掾支撑。
一座建筑物的钢构架设计是从屋顶向下进行的。
所有的荷载,不管是恒荷载还是活荷载(包括风荷载),都要按照连续水平面进行计算,直到每一根柱的荷载确定下来,并相应的对基础进行设计。
利用这些信息,结构设计师算出整个结构需要的钢构件的规格、形状,以及连接细节。
对于屋顶桁架和格构梁,设计师利用“三角剖分”的方法,因为三角形是唯一的固有刚度的结构。
背应力英文术语
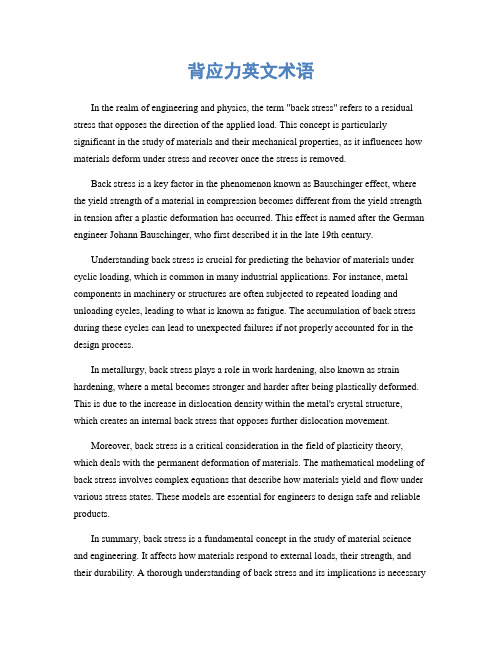
背应力英文术语In the realm of engineering and physics, the term "back stress" refers to a residual stress that opposes the direction of the applied load. This concept is particularly significant in the study of materials and their mechanical properties, as it influences how materials deform under stress and recover once the stress is removed.Back stress is a key factor in the phenomenon known as Bauschinger effect, where the yield strength of a material in compression becomes different from the yield strength in tension after a plastic deformation has occurred. This effect is named after the German engineer Johann Bauschinger, who first described it in the late 19th century.Understanding back stress is crucial for predicting the behavior of materials under cyclic loading, which is common in many industrial applications. For instance, metal components in machinery or structures are often subjected to repeated loading and unloading cycles, leading to what is known as fatigue. The accumulation of back stress during these cycles can lead to unexpected failures if not properly accounted for in the design process.In metallurgy, back stress plays a role in work hardening, also known as strain hardening, where a metal becomes stronger and harder after being plastically deformed. This is due to the increase in dislocation density within the metal's crystal structure, which creates an internal back stress that opposes further dislocation movement.Moreover, back stress is a critical consideration in the field of plasticity theory, which deals with the permanent deformation of materials. The mathematical modeling of back stress involves complex equations that describe how materials yield and flow under various stress states. These models are essential for engineers to design safe and reliable products.In summary, back stress is a fundamental concept in the study of material science and engineering. It affects how materials respond to external loads, their strength, and their durability. A thorough understanding of back stress and its implications is necessaryfor the development of advanced materials and the design of structures and components that can withstand the demands of modern engineering challenges.The study of back stress is not only limited to metals but also extends to polymers, composites, and other materials. Each material exhibits unique back stress characteristics, influenced by its microstructure, composition, and processing history. Researchers continue to explore the effects of back stress on new materials, seeking to enhance their performance for various applications.In the aerospace industry, for example, the control of back stress is vital for the development of lightweight yet strong materials that can endure the extreme conditions of flight. Similarly, in the automotive industry, understanding back stress contributes to the creation of vehicles that are both fuel-efficient and safe.In conclusion, back stress is a complex but essential aspect of material behavior. Its study and application are integral to the advancement of technology and the improvement of our daily lives through better, more resilient materials and products. As research progresses, our grasp of back stress and its management will continue to evolve, leading to innovations that will shape the future of engineering and design. 。
international journal of fatigue简介

international journal of fatigue简介International Journal of Fatigue (IJF) is a leading academic publication in the field of fatigue and structural integrity. With a focus on providing a platform for researchers and practitioners to share their knowledge and advancements, IJF plays a crucial role in disseminating cutting-edge research and promoting innovation in fatigue analysis and design.As one of the most respected journals in the field, IJF publishes high-quality papers that contribute to the understanding and prediction of fatigue behavior in various materials and structures. The journal covers a wide range of topics, including experimental investigations, theoretical studies, and numerical simulations related to fatigue and fracture mechanics.IJF welcomes submissions from both academia and industry, fostering collaboration between researchers and practitioners. The journal's editorial board comprises renowned experts from around the world, ensuring a rigorous and impartial peer-review process. This process ensures the quality and integrity of the published articles, making IJF a trusted source of information for scientists, engineers, and professionals in the field of fatigue.The scope of the journal encompasses several key areas, including fatigue crack growth, fatigue life prediction, fatigue testing techniques, and durability analysis. Additionally, IJF explores emerging topics such as multiscale fatigue modeling, environmental effects on fatigue, and fatigue in advanced materials and structures. By covering a broad range of subjects, IJF offers a comprehensive and up-to-date perspective on fatigue research.IJF aims to bridge the gap between fundamental research and practical applications. The journal encourages the submission of papers that have direct implications for industry, addressing real-world challenges and proposing practical solutions. By providing a platform for knowledge exchange, IJF facilitates the transfer of innovation from academia to industry, enabling technological advancements and enhancing the reliability and safety of engineering systems.In addition to regular research articles, IJF publishes review articles, technical notes, and case studies, further enriching the readers' experience and providing comprehensive insights into the field of fatigue. These diverse types of contributions enhance the journal's value as a reference for researchers, engineers, and practitioners seeking both in-depth analysis and practical advice.Furthermore, IJF embraces international collaboration and diversity, publishing articles from researchers around the globe. This global perspective ensures a wide range of perspectives and promotes the exchange of ideas and methodologies across different cultures and scientific communities.In conclusion, International Journal of Fatigue serves as a vital platform for the dissemination of knowledge and advancements in the field of fatigue and structural integrity. By fostering collaboration, promoting innovation, and providing a comprehensive perspective, IJF contributes significantly to the advancement of fatigue research and its practical applications. Researchers, engineers, and professionals can rely on IJF as a trusted sourceof high-quality research, helping them stay at the forefront of this critical field.。
ncode疲劳仿真操作流程

ncode疲劳仿真操作流程ENGLISH ANSWER.Introduction.Fatigue analysis is a critical aspect of engineering design, as it helps ensure the safety and durability of structures and components. nCode Fatigue is a powerful software tool that enables engineers to perform comprehensive fatigue simulations, taking into account various loading conditions, material properties, and environmental factors. This article provides a step-by-step guide to the nCode Fatigue simulation workflow, outlining the key steps involved in setting up, running, and analyzing fatigue simulations.Step 1: Model Setup.1. Import Geometry: Import the CAD geometry of the component or structure to be analyzed. nCode Fatiguesupports various CAD formats, including STEP, IGES, and Parasolid.2. Define Material Properties: Specify the material properties relevant to fatigue analysis, such as Young's modulus, Poisson's ratio, and fatigue strength coefficients.3. Create Load Cases: Define the loading conditionsthat the component will experience during its service life. Load cases can include static, dynamic, and environmental loads.4. Mesh the Model: Generate a mesh of the model using finite element analysis (FEA) techniques. The mesh density and element type should be carefully chosen to ensure accurate results.Step 2: Simulation Setup.1. Select Fatigue Criteria: Choose the fatigue criteria to be used in the simulation, such as the Goodman, Soderberg, or SWT criteria.2. Define Stress Concentration Factors: Identify any stress concentration areas in the model and apply appropriate stress concentration factors to account for their effects on fatigue life.3. Set Simulation Parameters: Specify the simulation parameters, including the number of cycles, time step, and convergence criteria.Step 3: Simulation Execution.1. Run the Simulation: Start the fatigue simulation and monitor its progress. nCode Fatigue utilizes advanced numerical algorithms to solve the fatigue equations and calculate fatigue damage accumulation.2. Monitor Results: Observe the simulation results in real-time, including the distribution of fatigue damage, stress, and strain.Step 4: Post-Processing.1. Analyze Fatigue Damage: Review the fatigue damage results and identify critical areas of the model that are prone to failure.2. Plot Damage Contours: Generate contour plots to visualize the distribution of fatigue damage throughout the model.3. Create Fatigue Life Estimates: Use the simulation results to estimate the fatigue life of the component under different loading conditions.Conclusion.By following these steps, engineers can leverage nCode Fatigue to perform comprehensive fatigue simulations, providing valuable insights into the fatigue behavior of their designs. This enables them to optimize designs for improved durability and safety, ensuring the reliability of their products in real-world applications.CHINESE ANSWER.简介。
土木专业英语unit3
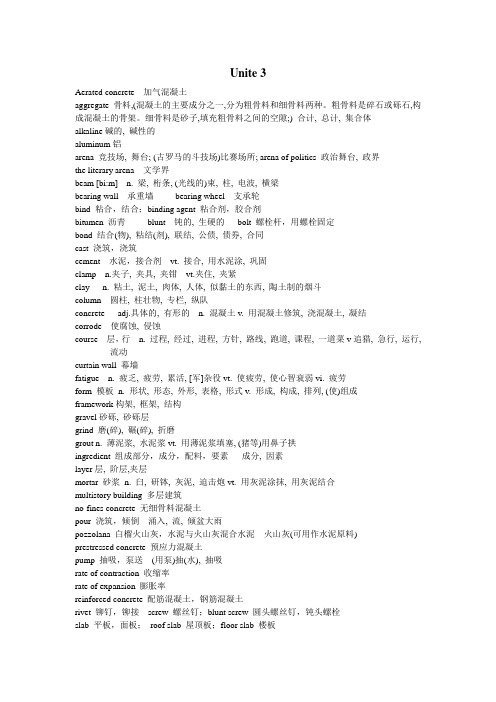
Unite 3Aerated concrete 加气混凝土aggregate骨料,(混凝土的主要成分之一,分为粗骨料和细骨料两种。
粗骨料是碎石或砾石,构成混凝土的骨架。
细骨料是砂子,填充粗骨料之间的空隙;) 合计, 总计, 集合体alkaline碱的, 碱性的aluminum铝arena 竞技场, 舞台; (古罗马的斗技场)比赛场所; arena of politics 政治舞台, 政界the literary arena 文学界beam [bi:m] n. 梁, 桁条, (光线的)束, 柱, 电波, 横梁bearing wall 承重墙bearing wheel 支承轮bind 粘合,结合;binding agent 粘合剂,胶合剂bitumen 沥青blunt 钝的, 生硬的bolt 螺栓杆,用螺栓固定bond 结合(物), 粘结(剂), 联结, 公债, 债券, 合同cast 浇筑,浇筑cement 水泥,接合剂vt. 接合, 用水泥涂, 巩固clamp n.夹子, 夹具, 夹钳vt.夹住, 夹紧clay n. 粘土, 泥土, 肉体, 人体, 似黏土的东西, 陶土制的烟斗column 圆柱, 柱壮物, 专栏, 纵队concrete adj.具体的, 有形的n. 混凝土v. 用混凝土修筑, 浇混凝土, 凝结corrode 使腐蚀, 侵蚀course 层,行n. 过程, 经过, 进程, 方针, 路线, 跑道, 课程, 一道菜v追猎, 急行, 运行, 流动curtain wall 幕墙fatigue n. 疲乏, 疲劳, 累活, [军]杂役vt. 使疲劳, 使心智衰弱vi. 疲劳form 模板n. 形状, 形态, 外形, 表格, 形式v. 形成, 构成, 排列, (使)组成framework构架, 框架, 结构gravel砂砾, 砂砾层grind 磨(碎), 碾(碎), 折磨grout n. 薄泥浆, 水泥浆vt. 用薄泥浆填塞, (猪等)用鼻子拱ingredient 组成部分,成分,配料,要素成分, 因素layer层, 阶层,夹层mortar 砂浆n. 臼, 研钵, 灰泥, 迫击炮vt. 用灰泥涂抹, 用灰泥结合multistory building 多层建筑no-fines concrete 无细骨料混凝土pour 浇筑,倾倒涌入, 流, 倾盆大雨pozzolana 白榴火山灰,水泥与火山灰混合水泥火山灰(可用作水泥原料)prestressed concrete 预应力混凝土pump 抽吸,泵送(用泵)抽(水), 抽吸rate of contraction 收缩率rate of expansion 膨胀率reinforced concrete 配筋混凝土,钢筋混凝土rivet 铆钉,铆接screw 螺丝钉;blunt screw 圆头螺丝钉,钝头螺栓slab 平板,面板;roof slab 屋顶板;floor slab 楼板span 跨度,孔距spray 喷射tarlike 像焦油的,焦油状的tensile 拉力的,受力的,抗拉的tensile strength 抗拉强度tension 拉力,拉伸thread 螺纹tile 瓦片,瓷砖;hollow tile 空心砖versatile 多用途的,万用的,通用的volcanic 火山的,火山口的;volcanic ash 火山灰weld 焊接1.2 the characters of grammar 语法特点(1)非人称的语气和客观态度,常使用it…结构专业英语多描述客观事物、现象和规律。
工业工程的专业英语词汇
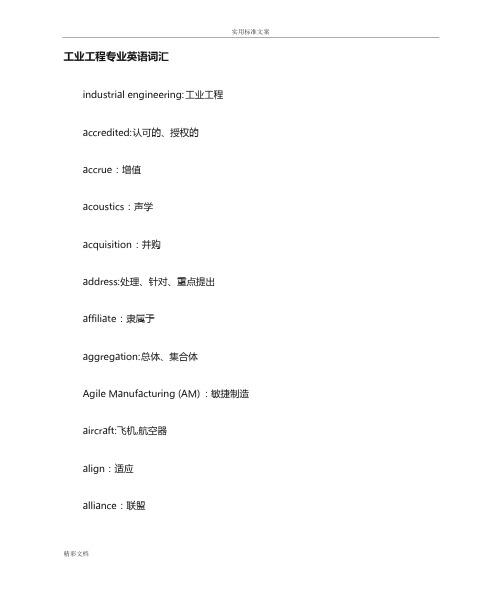
工业工程专业英语词汇industrial engineering:工业工程accredited:认可的、授权的accrue:增值acoustics:声学acquisition:并购address:处理、针对、重点提出affiliate:隶属于aggregation:总体、集合体Agile Manufacturing (AM):敏捷制造aircraft:飞机,航空器align:适应alliance:联盟ample:足够的、充裕的anatomical:解剖的ancillary:辅助的、附属的anthropometry:人体测量学appropriation: 占用artificial material:人工材料ASME: American Society of Mechanical Engineers:美国机械工程师协会assembly line:装配线assess:评估assiduity:勤奋、刻苦audit:审计automatic pallet changer:自动托盘转换装置automation:自动化ballistic:自然带弧形的bar code:条形码b atch p ro d uctio n:批量生产bench:工作台bill:清单bin:箱子biomechanical:生物力学的blade:刀片、叶片brand new:全新的b ud g e t-o rie nte d:面向预算的capacity:生产能力capital turnover:资金周转capital:资金carbon-filament:钨丝causal method:因果法cause and effect diagram:因果图cellular layout:单元式布局certification:认证change over :换模checksheet:检查表chronological:严密逻辑的chuck:卡盘circulate:循环、流通civil engineering:土木工程clamp:夹住closed loop:闭环CNC machine tools:计算机数控机床cockpit:飞机座舱、驾驶员座舱cognitive:认知的coil feeder:卷料进料器Co m m unicatio n Te chniq ue s in Lo g isticscompetitiveness:竞争力component:零件、组件、部件comprehensive interest:综合利益Computer Integrated Manufacturing Systems (CIMS):计算机集成制造系统computerized numerical controlconsecutive: 连续的continous improvement:持续改进continuous improvement:持续改进conveyor:输送机convoluted:复杂的、回旋的、弯曲的coordination:协调corkscrew: 螺丝刀co st-e ffe ctive:有成本效益的、划算的crank:曲柄critical examination technique:关键检测技术crossbar:十字杠culminate:达到顶点curricula: 课程(or curriculum)customer satisfaction:顾客满意cutback:缩减cylindrical:圆柱的prismatic:棱柱的dam:水坝decision-making:决策defective:有瑕疵的,有缺陷的definable:可定义的demonstrate:示范、说明dependent demand: 相关需求discipline:学科discrete:离散的dispersion:分散性distribution:配送、分销division:部门、分配、分开drill press:钻床drop delivery:堕送装置due date:交货期d ye:染料earning:收益、利润E-business:电子商务economic and knowledge-based era:知识经济时代economic batch quantity:经济批量economic globalization:经济全球化ECRS(eliminate combine rearrange simplify):取消、合并、重排、简化EDM: electron discharge machining:放电加工effectiveness:效果efficiency:效率ejector:斜槽、导轨electrical engineering:电气工程electricity: 电、电学、电流、电气electronic data interchange:电子数据交换E-Manufacturing:网络化制造engulf:吞没EOS:电子订货系统electronic ordering system ergonomically:工效学地ergonomics:工效学exaggerated:过大的、许多的explosion:爆炸法eyestrain:视觉疲劳,眼睛疲劳fabrication:制造facility:设备、设施factory layout:工厂布局family:簇fatigue:疲劳fatigue:疲劳feat:合适的feed grinding machine: 进给式磨床feedback:反馈feedback:反馈file:锉刀final product:最终产品fish bone diagram:鱼骨图fitness for use:适用性fixed position layout:定位布局fixture:固定设备、夹具flapped operation:节拍式加工flexible manufacturing system:柔性制造系统flow diagram:线路图flow process chart:流程程序图fluctuate: 波动forcible:强制的、有说服力的trunk:躯干torso forearm:前臂upper arm:大臂forecast:预测forge:锻造fo rg e:锻造formulate:阐述、制定fortification:防御工事forward-looking:有远见的fo und ry:铸造friction: 摩擦frustration:挫折fuel:燃料fully automated:全自动化gang process chart:联合程序图garment industry:制衣业gauge:计量器general packet radio service geographic information systems geometry:几何形状GIS:地理信息系统GPRS:通用分组无线业务GPS:全球定位系统global positioning system gravity feed:重力自流进料group technology:成组技术hand in hand :合作hard ware:硬件harmonious society:和谐社会haul: 拖、拉health-care delivery: 卫生保健服务high-tech:高科技hindrance:妨碍histogram:直方图hoist:起重机human factor:人因human-centered design:以人为中心设计hybrid layout:混合式布局hypotenuse:斜边(hypothesis:假设)identical:相同的idleness:空闲IE engineers:工业工程师(IEs)IE graduates:工业工程毕业生(IEs)impede:妨碍,阻止implicitly:隐含地incentive:鼓励inclined plane:斜面inclusive design:全方位设计inconsistency:不一致independent demand: 独立需求independent variable:自变量inevitable:不可避免的inspection:检测Institute of Industrial Engineers:工业工程师学会(IIE) instructor:讲师、教练instrument:仪器、器械intangible:无形的integrated equipment:集成设备interchangeability:互换性interface:界面、接口intermediary:中间人intermittent:间歇的internal combustion engine:内燃机International Accreditation Forum:国际认证论坛International Organization for Standardization:国际标准化组织(ISO)inventory control:库存控制Inventory:库存inventory:清单、库存invoicing:开发票item:物料项目jig:夹具job shop production:车间任务型生产judgment method:判断方法jumbled:混合的、混乱的knuckle:指关节wrist:腕关节elbow:肘关节lag:落后,延迟lathe:车床layout:布局lead time:提前期Lean Production (LP):精益生产literature:文献loading:装载locomotive:火车头logistics:物流long and short-term memory:长短时记忆lot for lot:批对批lot size:批量low-volume, high-variety production:多品种、小批量生产lubricant:润滑剂luggage:行李machine cell:机器单元machine tool:机床magnetism:磁学maintainability:可维护性maintaining:维护malfunction:故障manipulate:处理,使用,操纵man-machine process chart:人机程序图manufacturing industry:制造业manufacturing resources planning:制造资源计划market share:市场占有率master production scheduling:主生产计划material handling :物料搬运material requirements planning:物料需求计划mechanical engineering:机械工程mechanized:机械化的mental demand:脑力需求metal-working job shop :金工车间method study:方法研究methodology:方法metrics:度量m ilitary:军事的milling machine:铣床mission:使命、任务、目标MIT: 麻省理工学院Massachuse tts Institute o f Te chno lo g y molecular:分子的momentum:动量monetary:货币的、金融的morale:士气、纪律motion analysis:动作分析motion economy principles:动作经济原则motivation:激励multi-disciplinary:多学科性质的muscle:肌肉muscle:肌肉musculoskeletal disorder:肌骨失调navigation:导航netting:净需求计算normative:标准的notch: V型凹槽、切口nutrition:营养observe value:观察值offset:偏置法operation analysis:作业分析operation management:运作管理operation process chart: 工艺程序图opportunity:缺陷机会order fulfillment: 订单执行order lots:订单批量、订货量orient:定向otiose:无效的、多余的outlets:品牌直销购物中心overengineer:高于工程要求的package:包装pallet:托盘parameter:参数pareto chart:排列图part period cover:零件周期批量participation:参与partition:分割parts feeder:送料器physical science :自然科学(natural science)physiology:生理学pivot:轴、支点、中心点plot:以图的形式表示Pmts: predetermined motion time system:预定动作时间系统portable powered tool:便携式电动工具portray:描绘POS:销售时点系统point of sale systempositioning device:定位装置positioning:定位potentiality:潜能practitioner:开业者pre-assessment:预评估precondition :前提prediction:预言preliminary:预备的、初级的pre-positioned:预放在工作位置上proceed:行进、继续进行process analysis:程序分析process layout:工艺布局procurement:采购product layout:产品布局product life cycle: 产品生命周期production line:生产线production planning:生产计划production process:生产过程production scheduling:生产调度production system:生产系统productive:有生产价值的、多产的productivity :生产率profitability:收益率psychology:心理学pull production:拉动式生产Pythagorean theorem:勾股定理qualitative method:定性方法quality of conformance:符合性质量quality of design:设计质量quantitative method:定量方法rapid changeover:快速换模raw material:原材料rectangular:矩形的cube:立方体registrar:注册人员reliability:可靠性repetition:重复、复制品repetitive strain injury:重复性劳损replenishment:补充、补给re p ro ach:责备、谴责reputation:声誉requirement:需求reservation:预定resharpen:重磨retailer:零售商revenue:收入、税收RFID:无线射频技术radio frequency identificationrough cut capacity:粗能力计划saturation:饱和scatter diagram:散布图scheduling:调度、排程scheme:计划、设计screwdriver:螺丝刀seasonal patterns:季节模式semi-automatic(automated):半自动化sem inar:研讨班senso ry:感觉的service system:服务系统setup time:生产准备时间Shakespeare industry :莎士比亚产业sheet:薄板状的shroud:罩、遮蔽物simple lever:单杠杆simultaneously:同时地six sigma methodology: 六西格玛法socialize joint distribution:社会化共同配送specialization: 专业化specialty:专业specification:规范specs:规范、规格stamp:冲压standard data:标准资料standard deviation:标准偏差standardization: 标准化static electricity:静电学statistic:统计的statistical:统计学的steam engine:蒸汽机stock:库存sto re :仓库strategic planning:战略规划Stratfo rd-o n-Avo n, as we all kn o w, has only one Shake sp e are-b ut there are two distinctly se p arate and branches.subassembly:组件、部件substandard:低于标准的suite:软件包industry-William increasingly hostilesupply chain:供应链symmetrical:对称、匀称synchronous:同步的synthesize:综合tangible:有形的team spirit:团队精神Te chn ical Co m m itte e(TC)176:品质保证技术委员会template:模板template:模型thermal process:热处理thermal:热量的,热的third-party logistics:第三方物流threbligs:动素time study:时间研究time-series analysis:时间序列分析tolerance:容许偏差tote bin:搬运箱trade-off: 权衡transaction:业务、交易transformation:转换transmission:传送transportation:运输trivial:琐碎的tune:调整turbine:涡轮机、汽轮机two-hand process chart:双手程序图underengineer:低于工程要求的unloading:卸载unpredictable:不可预测的use r-ce nte re d:用户为中心的variable:变量vessel:管道vibration:振动vicinity:邻近visionary:远景warehouse:仓库warehouse:仓库、仓储weld:焊接wholesaler:批发商work measurement:作业测定work piece:工件work related upper limb disorder:工作引起的上肢功能障碍work sampling:工作抽样work unit:工件workhead:工作台、机台workholder:工件夹具wo rk-in-p ro ce ss:在制品workshop:车间、研讨会workstation:工作站。
随机振动疲劳分析流程
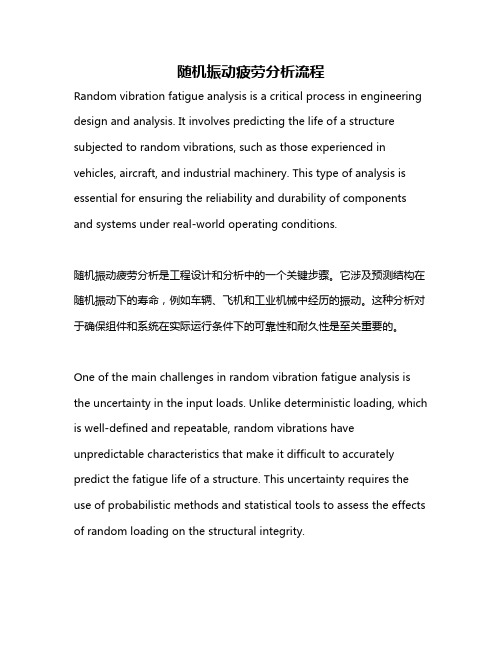
随机振动疲劳分析流程Random vibration fatigue analysis is a critical process in engineering design and analysis. It involves predicting the life of a structure subjected to random vibrations, such as those experienced in vehicles, aircraft, and industrial machinery. This type of analysis is essential for ensuring the reliability and durability of components and systems under real-world operating conditions.随机振动疲劳分析是工程设计和分析中的一个关键步骤。
它涉及预测结构在随机振动下的寿命,例如车辆、飞机和工业机械中经历的振动。
这种分析对于确保组件和系统在实际运行条件下的可靠性和耐久性是至关重要的。
One of the main challenges in random vibration fatigue analysis is the uncertainty in the input loads. Unlike deterministic loading, which is well-defined and repeatable, random vibrations have unpredictable characteristics that make it difficult to accurately predict the fatigue life of a structure. This uncertainty requires the use of probabilistic methods and statistical tools to assess the effects of random loading on the structural integrity.随机振动疲劳分析中的主要挑战之一是输入载荷的不确定性。
Fracture Mechanics and Fatigue

Fracture mechanics and fatigue are crucial concepts in the field of materials science and engineering. Fracture mechanics deals with the study of how materials behave when subjected to stress, particularly focusing on the initiation and propagation of cracks. On the other hand, fatigue is the phenomenon where materials fail due to repeated loading and unloading cycles, leading to the gradual growth of cracks until catastrophic failure occurs. Understanding these phenomena is essential for designing safe and reliable structures in various industries, such as aerospace, automotive, and civil engineering. One of the key aspects of fracture mechanics is the concept of stress intensity factor, which quantifies the stress field near the tip of a crack and predicts the likelihood of crack propagation. By analyzing the stress distribution around a crack, engineers can assess the critical conditions under which a crack will grow and ultimately lead to failure. This knowledge is crucial for determining the safety margins of structures and components, ensuring that they can withstand the expected loading conditions throughout their service life. Fatigue, on the other hand, poses a different challenge for materials engineers. Unlike static loading, fatigue loading involves cyclic stress variations that can lead to crack initiation and propagation even at stress levels below the material's ultimate strength. This phenomenon is particularly critical in applications where components are subjected to repeated loading, such as aircraft wings, automotive suspension systems, and offshore structures. By studying the fatigue behavior of materials, engineers can predict the fatigue life of a component and implement strategies to mitigate fatigue failure, such as improving material quality, introducing surface treatments, or redesigning the component geometry. In practice, fracture mechanics and fatigue analysis are often combined to assess the structural integrity of complex systems. Engineers use advanced modeling techniques, such as finite element analysis and computational simulations, to predict the behavior of materials under different loading conditions. By incorporating experimental data and theoretical models, engineers can optimize the design of structures and components to minimize the risk of failure due to fracture or fatigue. This integrated approach allows engineers to balance performance, cost, and safetyconsiderations in the design process, ensuring that structures meet the required standards and regulations. Despite the advancements in materials science and engineering, challenges still exist in predicting and preventing fracture and fatigue failures. The complex nature of material behavior, combined with the variability of loading conditions and environmental factors, makes it difficult to accurately predict the lifetime of structures and components. Moreover, unexpected failure events can have catastrophic consequences, leading to injuries, financial losses, and damage to the environment. As such, ongoing research and development efforts are focused on improving the understanding of fracture mechanics and fatigue behavior, developing new materials with enhanced durability and reliability, and implementing advanced testing and monitoring techniques to detect early signs of damage. In conclusion, fracture mechanics and fatigue are fundamental concepts in materials science and engineering that play a crucial role in ensuring the safety and reliability of structures and components. By studying the behavior of materials under different loading condition likelihood of crack initiation and propagation, assess the fatigue life of components, and optimize the design of structures to minimize the risk of failure. While challenges exist in predicting and preventing fracture and fatigue failures, ongoing research and development efforts are aimed at improving our understanding of material behavior and developing innovative solutions to enhance the durability and performance of engineering systems. By addressing these challenges, engineers can continue to push the boundaries of materials science and engineering, creating safer and more sustainable structures for the future.
冶金专业英语(全)
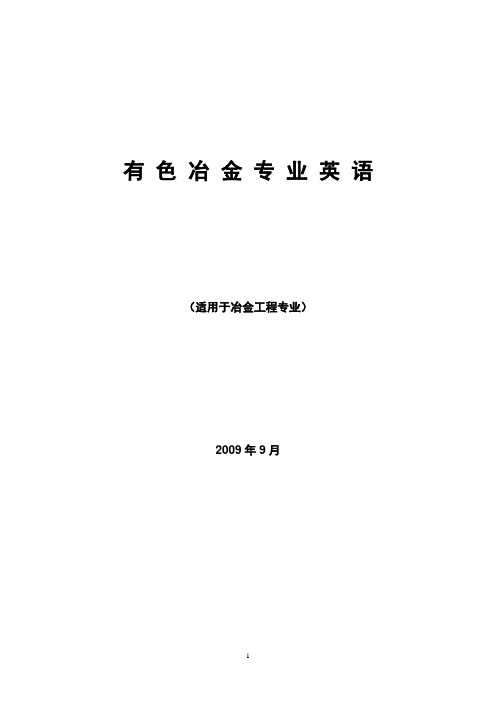
有色冶金专业英语(适用于冶金工程专业)2009年9月Lesson 3 Ore DressingOre dressing 选矿Concentrate v. 富积,浓缩,集聚n. 精矿,浓缩物Concentration n. 集中,浓缩,浓度Acid concentration 酸浓度Bulk n. 正体,主体,团块Gangue n. 脉石,尾矿,矿脉中的夹杂物Tailing n. 尾矿Severance n. 分离,隔离,碎散Beneficiation n. 分选Comminution n. 粉碎Run-of-mine n. 原矿Middling n. 中矿Liberation n. 解离Crush n. v. 粉碎,碾碎,挤压Grind n. v. 研磨,磨细Screen n. v. 筛,筛分Jigging n. 跳选,跳汰选Hand picking 手选Luster n. 光泽,光亮v. 闪光,发光Specific gravity 比重Magnetic permeability 磁导率Inductive charging 感应电荷Electrostatic separation 静电分离Fracture n. 断口,裂缝Automatic sorting of radioactive natures放射性自动选矿Magnitude n. 大小,尺寸,量级,强度,等级Magnetic separation 磁选Magnetic field 磁场Gravity concentration 重力选矿Medium n. 介质,媒介,中间物,培养基Dilate v. (使)膨胀,扩张,扩大Dilated bed 松散床层Dilation n. 膨胀系数,传播,伸缩,蔓延Lip n. 凸出部分,唇部Diverse adj. 不同的,互异的,各种各样的Table n. 摇床,淘汰盘Tabling 摇床选,淘汰选Motion n. 运动,输送,行程,机械装置,运动机构Sink-float separation 重介质分选Suspension n. 悬浮物,悬浮液Cone n. 圆锥体,锥形漏斗,圆锥破碎机Stir n. v. 移动,摇动,搅拌Stirrer n. 搅拌器,搅拌机Rotary adj. 旋转的,回转的,转动的Circumference n. 圆周,周边Rotating motion 旋转装置,旋转设备Floatation n. 浮选Pulp n. 矿浆,浆料v. 制浆,浆化Sluice n. 槽,排水道,水槽Froth floatation 泡沫浮选Hematite n. 赤铁矿Pyrolusite n. 软锰矿Diamond n. 金刚石Graphite n. 石墨Ore dressing concerns with the technology of treatment of ores to concentrate their valuable constituents (minerals) into products (concentrate) of smaller bulk, and simultaneously to collect the worthless material (gangue) into discardable waste (tailing). The fundamental operations of ore-dressing processes are the breaking apart of the associated constituents of the ore by mechanical means (severance) and the separation of the severed components (beneficiation) into concentrate and tailing, using mechanical or physical methods which do not effect substantial chemical changes.1Severance. Comminution is a single, or multistage processes whereby ore is reduced from run-of-mine size to that size needed by the beneficiation processes. The process is intended to detailed control, a class of particles containing both mineral and gangue (middling particles) are also produced. The smaller the percentage of middling the greater the degree of liberation. Comminution is divided into crushing (down to 6-to 14-mush) and grinding (down to micron size). Crushing is usually done in three stages: coarse crushing from run-of-mine size to 4-6 in., or coarser; intermediate crushing down to about 1/2 in.; and fine crushing to 1/4 in. or less. Screen is a method of sizing whereby graded products are produced, the individual particles in each grade being of nearly the same size. In beneficiation, screening is practiced for two reasons: as and integral part of the separate on process, for example, in jigging, and to produce a feed of such size range as is compatible with the applicability of the separation process.Beneficiation. This step consists of two fundamental operations: the determination that an individual particle is either a mineral or a gangue particle (selection); and the movement of selected particles via different paths (separation) into the concentrate and tailing products.2 When middling particles occur, they will either be selected according to their mineral content and then caused to report as concentrate or tailing, or be separated as a third product (middling).3 In the latter case, the middling is reground to achieve further liberation, and the product is fed back into the stream of material being treated.Selections based upon some physical or chemical property in which the mineral and gangue particles differ in kind or degree or both. Thus in picking, the old form of beneficiation, color, luster, and shape are used to decide whether a lump of ore is predominantly mineral or gangue. Use is made of differences in other physical or chemical properties, such as specific gravity, magnetic permeability, inductive charging (electrostatic separation), surface chemical properties, bulk chemical properties, weak planes of fracture (separation by screening), and gamma-ray emission (automatic sorting of radioactive nature).Separation is achieved by subjecting each particle of the mixture to a set of forces that is usually the same irrespective of the nature of the particles excepting for the force based upon the discriminating property. This force may be present for both mineral and gangue particles but differing in magnitude, or it may be present for one type of particle and absent for the other. As a result of this difference separation ispossible, and the particles are collected as concentrate or tailing.Magnetic separation utilizes the force exerted by a magnetic field upon magnetic materials to counteract partially or wholly the effect of gravity. Thus under the action of these two forces different paths are produced for the magnetic and nonmagnetic particles.Gravity concentration is based on a discriminating force, the magnitude of which varies with specific gravity. The other force that is usually operating in gravity methods is the resistance to relative motion exerted upon the particles by the fluid or semi-fluid medium in which separation takes place Jigging is a gravity method that separates mineral from gangue particle by utilizing an effective difference in settling rate through a periodically dilated bed. During the dilation heavier particles work their way to the bottom while the lighter particles remain on top and are discharged over the lip. Jigging is practiced on materials that are liberated upon being reduced to sizes ranging from 3/2 in., down to several millimeters. It has been used on such diverse ores as coal, iron ores, gold and lead ores.Tabling is a gravity method in which the feed, introduced onto an inclined plane and reciprocated deck, moves in the direction of motion while simultaneously being washed by a water film which moves it also at right angles to the motion of the deck.4 The heavier mineral and the lighter gangue are usually collected over the edges of the deck. The boundary between the heavier mineral and lighter gangue particles is roughly a linear diagonal band on the deck of the table. This diagonal band is not stationary; rather it tends to move about a mean position. In practice therefore, a third product, the middling, is collected between the discharge edges of concentrate and gangue. If the feed to the table has been crushed or ground to produce liberation, then the middling is returned to the feed. If liberation has not been achieved, the middling is returned to the crushing-grinding section of the mill. Tables may be used to treat relatively coarse material (sand tables) with sizes ranging from about 2~3 mm down to 0.07 mm.Sink-float separation is the simplest gravity method and is based on existing differences in specific gravity. The feed particles are introduced into a suspension, the specific gravity of which is between that of the mineral and gangue particles, with the result that particles of higher specific gravity sink while those of lower specific gravity float.5The separator is a cone equipped with a slowly operated stirrer which serves to impart slow rotary motion to the suspension and prevent the suspension from settling out on the walls. Feed is introduced at one point of the circumference and is slowly moved by the rotating motion of the suspension. By the time this material has reached the discharge point on the circumference, those particles whose specific gravity is greater than that of the suspension have moved down through the suspension so that only float particles are discharged at the top, the sink particles are discharged at the bottom.Flotation is used to separate valuable minerals from waste rock or gangue, in which the ground ore is suspended in water and, after chemical treatment, subjected to bubbles of air. The minerals that are to be floated attach to the air bubbles, rise through the suspension, and are removed with the froth that forms on top of the pulp. Froth flotation was first used to recover sulfide minerals that were too fine to be recovered by gravity concentrators such as jigs, tables, and sluices. Froth flotation is also used to concentrate oxide minerals such as hematite (Fe2O3) and pyrolusite (MnO2), and native elements such as sulfur, silver, gold, copper and carbon (both graphite and diamond). Froth flotation is also used to separate the silicate minerals.Lesson 5 Materials Science and EngineeringEmbrace 包括Ceramics 陶瓷Inanimate 无生命的Homogeneous 均匀的Predominate 主导Rigidity 刚性Weldability 可焊性Composite 复合材料Spectrum 种类Brass 黄铜Bronze 青铜Invar 因钢(NiFe) Cement 水泥Ferrite 铁素体Garnet 石榴石PVC 聚氯乙烯Polyethylene 聚乙烯PTFE 聚四氟乙烯Terylene 涤纶nylon 尼龙leather 皮革reinforced 增强dispersion 弥散supersonic 超声波optimum 最优fabrication 人工制作invariable 不变的corrosion 腐蚀fatigue 疲劳assess 评估1. Materials Science“Materials Science” is a subject for engineers of the modern age. It embraces a study of different materials regarding their structures, properties and uses. The “material” here does not refer to all matter in the Universe. If this were so, it would include all the physical sciences and the life sciences form astronomy to zoology. We can restrict the definition only to matter useful to mankind. Even here, the range is too broad for the purposes of the engineer. For example, we can list a large number of things useful, to man, such as food, medicines, explosives, chemicals, water, steel, plastics and concrete, only a few of which qualify as engineering materials. We have then to be more specific, and define materials as that part of inanimate matter that is useful to the engineer in the practice of his profession.1Recently the term, materials refer only to solid materials, even though it is possible to quote a number of examples of liquid and gaseous materials such as sulfuric acid and steam, which are useful to the engineer.The word ‘science’ refers to the physical science, in particular to chemistry and physics. As we confine ourselves mainly to solid in material science, the subject is related to solid state chemistry and solid state physics. The engineering usefulness of the matter under study is always deep in mind. In this respect,material ceramics science comes heavily from the engineering sciences such as metallurgy, and polymer science. These, in their own time, have grown out of their interaction with the basic sciences of chemistry and physics.Therefore, Material Science refers to that branch of applied science concerned with investigating the relationship existing between the structure of materials and their properties, and it concerns with the interdisciplinary study of materials for entirely practical purposes.2 Material science has developed rapidly during the last ten years. The new approach of material science has paid of handsomely in many ways and they have solved the problems in selection of right materials in complex situations.2. Classes of Engineering MaterialsWithin the scope of material science, the engineering materials may be classified in three broad groups according to their mode of occurrence:(1) Metals and alloys(2) Ceramics(3) Organic polymers.A metal is an elemental substance. An alloy is a homogeneous mixture of two or more metals or a metal and nonmetal. Among the solid materials, metals and alloys predominate because of their useful characteristics of hardness, strength, rigidity, formability, machinability, weldability, conductivity and dimensional stability.Ceramics are materials consisting of phases. A phase is a physically separable and chemically homogeneous constituent of a material. These are themselves compounds of metallic and non-metallic elements. All metallic compounds, rocks minerals, glass, glass-fiber, abrasives and all fired clays are ceramics.Organic materials are those materials derived directly from carbon. They usually consist of carbon chemically combined with hydrogen, oxygen or other nonmetallic substances, and their structures are, in many instances, fairly complex. Plastics and synthetic rubbers are common organic engineering materials.Table 1 shows a broad spectrum of engineering materials which shows not only typical examples from each of these three groups but also gives a number of examples of materials which are composite up of two groups.3 In general, in each and every engineering application we find material from all the three basic types of materials described above.Table 1. Some important grouping of materialsSince the engineer must specify the materials for TV sets, computers, suspension bridges, oil refineries, rocket motors, nuclear reactors, or supersonic transports he must have sufficient knowledge to select the optimum material for each application. Although experience provides the engineer with a starting point for selection of materials, the skill of the engineer will be limited unless he understands the factors that contribute to the properties of materials.43. Selection of MaterialsRight type of material is to be selected for a particular type of work. The selections of the right materials for given requirements, the proper use of those materials, development of new ways of using them for greater effectiveness, all are direct responsibility of the engineer.To fulfill this responsibility, the engineer must have a thorough knowledge of the nature and behavior of materials. The study of the nature of materials has its foundation in chemistry and physics and that of behavior of materials involves the application, of the principles of the nature of materials, under the varied conditions found in engineering practice.3 This behavior of materials is determined by composition, structure, service conditions, and the interactions among them. All materials have limitations within whichthey perform well but beyond which they cannot be used satisfactorily.However, the selection of a material for a specific application is invariably a thorough, lengthy, and expensive investigation. Almost always more than one material is suited to the application, and the final selection is a compromise that weights the relative advantages and disadvantages. The varied requirements to three broad demands: (1) Service requirements; (2) Fabrication requirements; (3) Economic requirements.The service requirements have important role in material selection. The material must stand up to service demands. Such demands commonly include dimensional stability, corrosion resistance, adequate strength, hardness, and toughness, heat resistance. In addition to any such basic requirements, other properties may be required such as a low electrical resistance, high or low heat conductivity, fatigue resistance, or others.Fabrication requirements are also to be considered in material selection. It must be possible to shape the material, and to join it to other material. The assessment of fabrication requirements concerns questions of machinability, hardenability, heat treatability, ductility, castability, and weldability, qualities that are sometime quite difficult to assess.Along with the above two requirements, the economic requirements give final shape in material selection. Goods must be produced at lower cost. The object is the minimum over all cost of the component to be made, and this objective is sometimes attained only by increasing one or more of the cost componentsLesson 6 MetallurgyMetallurgy n. 冶金,冶金学Non-ferrous metallurgy 有色冶金学Chlorine metallurgy 氯化冶金学Powder metallurgy 粉末冶金学Extractive metallurgy 提取冶金学Meteoric iron 陨铁Craftsmanship n. 手艺,技能Craftsman n. 技工,工匠Ornamental adj. 装饰用的,观赏的n. 装饰品Metalworking n. 金属加工Ceremonial adj. 正式的,礼仪的,仪式的Decorative adj. 装饰的Decorative arts 装饰艺术Cast n. v. 铸造,铸件Process metallurgy 过程冶金Production metallurgy 生产冶金Physical metallurgy 物理冶金Chemical metallurgy 化学冶金Mechanical metallurgy 机械冶金,力学冶金Unit operation 单元操作Unit process 单元过程Flux n. 熔剂Solvent n. 溶剂Slag n. 渣,炉渣v. 造渣Electrolyte n. 电解质,电解液Depletion n. 用尽,消耗,贫化,提取金属Deposit n. v. 沉积,沉淀,电积Blast furnace 鼓风炉,高炉Crude iron 生铁crystal structure 晶体结构neutron n. 中子diffraction n. 衍射crystal imperfection 晶体缺陷plastic deformation 塑性变形metallography n. 金相学microscopy n. 显微镜学,显微技术forging n. 锻造,锻件blowhole n. 气孔thermodynamics n. 热力学kinetics n. 动力学Steelmaking n. 炼钢Scrape n. 废料Leach v. 浸出,溶出Electrochemical reduction cell 电化学还原电池Inorganic chemistry 无机化学Pyro-metallurgy 火法冶金Hydro-metallurgy 湿法冶金elevated temperature 高温reduce v. 还原reduction n. 还原charcoal n. 木炭,炭spontaneous adj. 天然的,自动的,自发的residue n. 残渣,剩余物,残余物,炉渣roasting n. 焙烧pig iron 粗铁,生铁refine v. n. 精炼,提纯,纯化uranium n. 铀tungsten n. 钨molybdenum n. 钼isolate v. 隔离,隔绝,切断recovery n. 回收,回收率,回复,恢复scope n. 范围,领域,目标revert n. 返料metalloid n. 类金属adj. 类金属的selenium n. 硒tellurium n. 碲amenability n. 可控制性,可处理性adaptability n. 适应性hafnium n. 铪zirconium n. 锆flexibility n. 适应性,灵活性Metallurgy is the science of metallic materials. Metallurgy as a branch of engineering is concerned with the production of metals and alloys, their adaptation to use, and their performance in service. As a science, metallurgy is concerned with the chemical reactions involved in the processes by which metals are produced and the chemical, physical, and mechanical behavior of metallic materials.1Metallurgy has played an important role in the history of civilization. Metals were first produced more than 6000 year age. Because only a few metals, principally gold, silver, copper and meteoric iron, occur in the uncombined state in nature, and then only in small quantities, primitive metallurgists had to discover ways of extracting metals from their ores. Fairly large-scale production of some metals was carried out with technical competence in early Near Eastern and Mediterranean civilizations and in the Middle Ages in central and northern Europe. Basic metallurgical skills were also developed in other parts of the world.The winning of metals would have been of little value without the ability to work them. Great craftsmanship in metalworking developed in early times; the objects produced included jewelry, large ornamental and ceremonial objects, tools and weapons. It may be noted that almost all early materials and techniques that later had important useful applications were discovered and first used in the decorative arts.2 In the Middle Ages metalworking was in the hands of individual or groups of craftsmen. The scale and capabilities of metalworking developed with the growth of industrial organizations. Today’s metallurgical plants supply metals and alloys to the manufacturing and construction industries in many forms such as beams, plates, sheets, bars, wire, and castings. Rapidly developing technologies such as communications, nuclear power, and space exploration continue to demand new techniques of metal production and processing.The field of metallurgy may be divided into process metallurgy, (production metallurgy, extractive metallurgy) and physical metallurgy. According to another system of classification, metallurgy comprises chemical metallurgy, mechanical metallurgy (metal processing and mechanical behavior in service), and physical metallurgy. The more common division into process metallurgy and physical metallurgy, which isadopted here, classifies metal processing as a part of process metallurgy and the mechanical behavior of metals as a part of physical metallurgy.Process metallurgy Process metallurgy, the science and technology used in the productions of metals, employs some of the unit operations and unit processes as chemical engineering. These operations and processes are carried out with ores, concentrates, scrap metals, fuels, fluxes, slag, solvents, and electrolytes. Different metal adopts different combinations of operations and processes, but typically the production of a metal involves two major steps. The first is the production of an impure metal from ore minerals, commonly oxides or sulfides, and the second is the refining of the reduced impure metal, for example, by selective oxidation of impurities or by electrolysis. Process metallurgy is continually challenged by the demand for metals that have not been produced previously or are difficult to produce; by the depletion of the richer and more easily processed ores of the traditional metals; and by the need for metals of greater purity and higher quality. The mining of leaner ores has greatly enhanced the importance of ore dressing methods for enriching raw materials for metal production. Several nonferrous metals are commonly produced from concentrates. Iron ores are also increasingly treated by ore dressing.Process metallurgy today mainly involves large scale operations. A single blast furnace produces crude iron at the rate of 3,00~11,000 tons per day. A basic oxygen furnace for steelmaking consumes 800 tons of pure oxygen together with required amounts of crude iron and scrap to produce 12,000 tons of steel per day. Advanced methods of process analysis and control are now being applied to such processing system. The application of vacuum to extraction and refining processes, the leaching of low-grade ores for the extraction of metals, the use of electrochemical reduction cells, and the refining of reactive metals by processing through the vapor state are other important developments.Because the production of metals employs many different chemical reactions, process metallurgy has been closely associated with inorganic chemistry. Techniques for analyzing ores and metallurgical products originated several centuries ago and represented an early stage of analytical chemistry. Application of physical chemistry to equilibrium and kinetics of metallurgical reactions has led to great progress in metallurgical chemistry.According to temperature at which the process is carried out process metallurgy may be divided into pyrometallurgy and hydrometallurgy. Pyrometallurgy is processes employing chemical reactions at elevated temperatures for the extractions of metals from ores and concentrates. The use of heat to cause reduction of copper ores by charcoal dates from before 3,000 B.C. The techniques of pyrometallurgy have been gradually perfected as knowledge of chemistry has grown and as sources of controlled heating andmaterials of construction for use at high temperature have become available.3Pyrometallurgy is the principal means of metal production.The advantages of high temperature for metallurgical processing are several: chemical reaction rates are rapid, reaction equilibriums change so that processes impossible at low temperature become spontaneous at higher temperature, and production of the metal as liquid or gas facilitates physical separation of metal from residue.4The processes of pyrometallurgy may be divided into preparation processes which convert the raw material to a form suitable for further processing (for example, roasting to convert sulfides to oxides), reduction processes which reduce metallic compounds to metal (the blast furnace which reduces iron oxide to pig iron), and refining processes which remove impurities from crude metal (fractional distillation to remove iron, lead, and cadmium from crude zinc).The complete production scheme, from ore to refined metal, may employ pyrometallurgical processes (steel, lead, tin, zinc), or only the primary extraction processes may be pyrometallurgical, with other methods used for refining (copper, nickel). 5 In some case (uranium, tungsten, molybdenum), isolated pyrometallurgical processes are used in a treatment scheme that is predominately nonpyrometallurgical.Hydrometallurgy is the extraction and recovery of metals from their ores by processes in which aqueous solutions play predominant role. Two distinct processes are involved in hydrometallurgy; putting the metal values in the ore into solution via the operation known as leaching; and recovering the metal values from solution, usually after a suitable solution purification or concentration step, or both. The scope of hydrometallurgy is quite broad and extends beyond the processing of ores to the treatment of metal concentrates, metal scrap and revert materials, and intermediate products in metallurgical processes. Hydrometallurgy enters into the production of practically all nonferrous metals and or metalloids, such as selenium and tellurium.The advantages of hydrometallurgy are applicability to low-grade ores (copper, uranium, gold, silver), amenability to the treatment of materials of quite different compositions and concentrations, adaptability to separation of highly similar materials (hafnium from zirconium), flexibility in terms of the scale of operations, simplified materials handling as compared with pyrometallurgy, and good operational and environmental control.Physical metallurgy investigates the effects of composition and treatment on the structure of metal and the relations of the structure to the properties of metals. Physical metallurgy is also concerned with the engineering applications of scientific principles to the fabrication, mechanical treatment, heat treatment, and service behavior of metals.The structure of metals consists of their crystal structure, which is investigated by x-ray, electron, and neutron diffraction, their microstructure, which is the subject or metallography, and their macrostructure. Crystal imperfections provide mechanisms for processes occurring in solid metals, for example, the movement of dislocations results in plastic deformation. Crystal imperfections are investigated by x-ray diffraction and metallographic methods, especially electron microscopy. The microstructure is determined by the constituent phases and the geometrical arrangement of the microcrystals (grains) formed by those phases. Macrostructure is important in industrial metals. Phase transformations occurring in the solid state underlie many heat-treatment operations. The thermodynamics and kinetics of these transformations are a major concern of physical metallurgy. Physical metallurgy also investigates changes in the structure and properties resulting from mechanical working of metals.Lesson 12 Calcination and RoastingCalcination n. 焙烧,煅烧 calcine 焙砂 Decomposition n. 分解,裂解 Metal hydrate 金属氢氧化物 Carbonate n. 碳酸盐 Basic sulphate 碱式硫酸盐 Rotary kiln 回转窑 Shaft furnace 竖炉 Dead roasting 死烧 Sulphating roasting 硫酸化焙烧 Reduction roasting 还原焙烧 equillibrium constant 平衡常数 kellog diagram 凯洛格相图 predominance n. 优势,优越 predominance area 优势区 partial roasting 部分焙烧 selective roasting 选择性焙烧 chloridizing roast 氯化焙烧 smelt n. v. 熔炼noble adj. 贵重的,惰性的noble metal 惰性金属,贵金属 hypothetical adj. 假定的,有前提的 fume n. 烟气halide n. 卤化物volatilizing roast 挥发焙烧 magnetizing roast 磁化焙烧magnetite n. 磁铁矿flash roaster 闪速焙烧炉,飘悬焙烧炉 inject v. 喷射,喷入fluidise v. 流态化fluidized bed roaster 流态化焙烧炉 burner n. 喷嘴suspend v. 悬浮,漂浮fluo-solids roaster 流化-闪速焙烧炉 matte n. 冰铜,锍reverberatory furnace 反射炉1. CalcinationCalcination involves the chemical decomposition of the mineral and is achieved by heating to above the mineral’s decom position temperature (T D ) or by reducing the partial pressure of the gaseous product (P H 2O , P CO 2) below that of its equilibrium partial pressure for a certain constant temperature.1 For example,CaCO 3 = CaO + CO 2T D = 900℃ (under standard thermodynamic conditions)Calcination is mainly used to remove water, CO 2 and other gases which are chemically bound in metal hydrate and carbonates as these minerals have relatively low decomposition temperatures.2Calcinations are conducted in rotary kilns, shaft furnaces or fluidized bed furnaces.2. Roasting of metal concentratesThe most important roasting reactions are those concerning metal sulfide concentrates and involve chemical combination with the roasting atmosphere.Possible reactions include:MS + 3O 2 = 2MO + 2SO 2 (dead roast)MS + 2O 2 = MSO 4 (sulfating roast)MS + O 2 = M + SO 2 (reduction roast)Other equilibria which need to be taken into account include:(1/2)S 2 + O 2 = SO 2 andSO 2 + (1/2)O 2 = SO 3。
疲劳寿命的计算流程
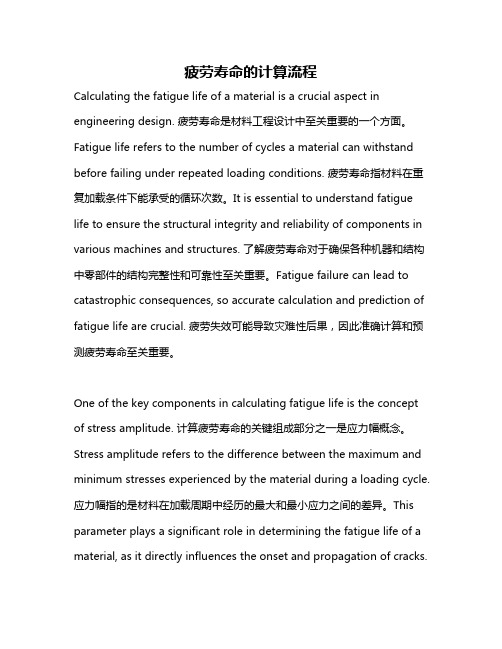
疲劳寿命的计算流程Calculating the fatigue life of a material is a crucial aspect in engineering design. 疲劳寿命是材料工程设计中至关重要的一个方面。
Fatigue life refers to the number of cycles a material can withstand before failing under repeated loading conditions. 疲劳寿命指材料在重复加载条件下能承受的循环次数。
It is essential to understand fatigue life to ensure the structural integrity and reliability of components in various machines and structures. 了解疲劳寿命对于确保各种机器和结构中零部件的结构完整性和可靠性至关重要。
Fatigue failure can lead to catastrophic consequences, so accurate calculation and prediction of fatigue life are crucial. 疲劳失效可能导致灾难性后果,因此准确计算和预测疲劳寿命至关重要。
One of the key components in calculating fatigue life is the concept of stress amplitude. 计算疲劳寿命的关键组成部分之一是应力幅概念。
Stress amplitude refers to the difference between the maximum and minimum stresses experienced by the material during a loading cycle. 应力幅指的是材料在加载周期中经历的最大和最小应力之间的差异。
fatigue
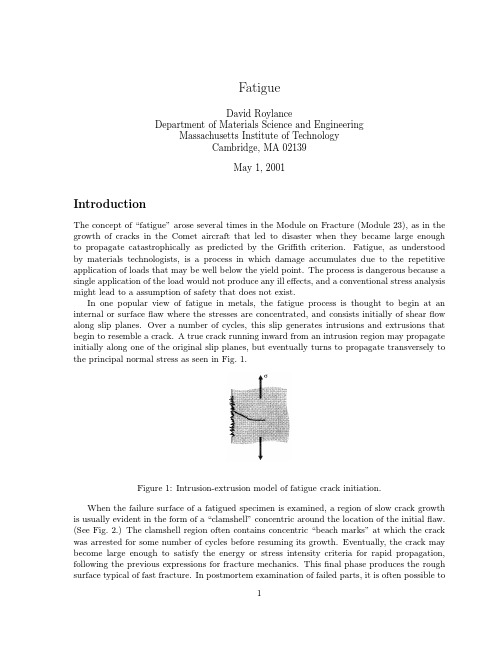
FatigueDavid RoylanceDepartment of Materials Science and EngineeringMassachusetts Institute of TechnologyCambridge,MA02139May1,2001IntroductionThe concept of“fatigue”arose several times in the Module on Fracture(Module23),as in the growth of cracks in the Comet aircraft that led to disaster when they became large enough to propagate catastrophically as predicted by the Griffith criterion.Fatigue,as understood by materials technologists,is a process in which damage accumulates due to the repetitive application of loads that may be well below the yield point.The process is dangerous because a single application of the load would not produce any ill effects,and a conventional stress analysis might lead to a assumption of safety that does not exist.In one popular view of fatigue in metals,the fatigue process is thought to begin at an internal or surfaceflaw where the stresses are concentrated,and consists initially of shearflow along slip planes.Over a number of cycles,this slip generates intrusions and extrusions that begin to resemble a crack.A true crack running inward from an intrusion region may propagate initially along one of the original slip planes,but eventually turns to propagate transversely to the principal normal stress as seen in Fig.1.Figure1:Intrusion-extrusion model of fatigue crack initiation.When the failure surface of a fatigued specimen is examined,a region of slow crack growth is usually evident in the form of a“clamshell”concentric around the location of the initialflaw. (See Fig.2.)The clamshell region often contains concentric“beach marks”at which the crack was arrested for some number of cycles before resuming its growth.Eventually,the crack may become large enough to satisfy the energy or stress intensity criteria for rapid propagation, following the previous expressions for fracture mechanics.Thisfinal phase produces the rough surface typical of fast fracture.In postmortem examination of failed parts,it is often possible tocorrelate the beach marks with specific instances of overstress,and to estimate the applied stress at failure from the size of the crack just before rapid propagation and the fracture toughness of the material.Figure2:Typical fatigue-failure surfaces.From B.Chalmers,Physical Metallurgy,Wiley,p.212, 1959.The modern study of fatigue is generally dated from the work of A.W¨o hler,a technologist in the German railroad system in the mid-nineteenth century.Wohler was concerned by the failure of axles after various times in service,at loads considerably less than expected.A railcar axle is essentially a round beam in four-point bending,which produces a compressive stress along the top surface and a tensile stress along the bottom(see Fig.3).After the axle has rotated a half turn,the bottom becomes the top and vice versa,so the stresses on a particular region of material at the surface varies sinusoidally from tension to compression and back again.This is now known as fully reversed fatigue loading.Figure3:Fatigue in a railcar axle.S-N curvesWell before a microstructural understanding of fatigue processes was developed,engineers had developed empirical means of quantifying the fatigue process and designing against it.Perhaps the most important concept is the S-N diagram,such as those shown in Fig.41,in which a constant cyclic stress amplitude S is applied to a specimen and the number of loading cycles N until the specimen fails is lions of cycles might be required to cause failure at lower loading levels,so the abscissa in usually plotted logarithmically.Figure4:S−N curves for aluminum and low-carbon steel.In some materials,notably ferrous alloys,the S−N curveflattens out eventually,so that below a certain endurance limitσe failure does not occur no matter how long the loads are cycled.Obviously,the designer will size the structure to keep the stresses belowσe by a suitable safety factor if cyclic loads are to be withstood.For some other materials such as aluminum,no endurance limit exists and the designer must arrange for the planned lifetime of the structure to be less than the failure point on the S−N curve.Statistical variability is troublesome in fatigue testing;it is necessary to measure the lifetimes of perhaps twenty specimens at each of ten or so load levels to define the S−N curve with statistical confidence2.It is generally impossible to cycle the specimen at more than approxi-mately10Hz(inertia in components of the testing machine and heating of the specimen often become problematic at higher speeds)and at that speed it takes11.6days to reach107cycles of loading.Obtaining a full S−N curve is obviously a tedious and expensive procedure.Figure5:Variability in fatigue lifetimes and fracture strengths.Atfirst glance,the scatter in measured lifetimes seems enormous,especially given the log-arithmic scale of the abscissa.If the coefficient of variability in conventional tensile testing is usually only a few percent,why do the fatigue lifetimes vary over orders of magnitude?It must be remembered that in tensile testing,we are measuring the variability in stress at a given number of cycles(one),while in fatigue we are measuring the variability in cycles at a given stress.Stated differently,in tensile testing we are generating vertical scatter bars,but in fatigue they are horizontal(see Fig.5).Note that we must expect more variability in the lifetimes as the S−N curve becomesflatter,so that materials that are less prone to fatigue damage require more specimens to provide a given confidence limit on lifetime.Effect of mean loadOf course,not all actual loading applications involve fully reversed stress cycling.A more general sort of fatigue testing adds a mean stressσm on which a sinusoidal cycle is superimposed,as shown in Fig.6.Such a cycle can be phrased in several ways,a common one being to state the alternating stressσalt and the stress ratio R=σmin/σmax.For fully reversed loading,R=−1.A stress cycle of R=0.1is often used in aircraft component testing,and corresponds to a tension-tension cycle in whichσmin=0.1σmax.Figure6:Simultaneous mean and cyclic loading.A very substantial amount of testing is required to obtain an S−N curve for the simple case of fully reversed loading,and it will usually be impractical to determine whole families of curves for every combination of mean and alternating stress.There are a number of strategems forfinessing this difficulty,one common one being the Goodman diagram.shown in Fig.7.Here a graph is constructed with mean stress as the abscissa and alternating stress as the ordinate, and a straight“lifeline”is drawn fromσe on theσalt axis to the ultimate tensile stressσf on the σm axis.Then for any given mean stress,the endurance limit—the value of alternating stress at which fatigue fracture never occurs—can be read directly as the ordinate of the lifeline at that value ofσm.Alternatively,if the design application dictates a given ratio ofσe toσalt,a line is drawn from the origin with a slope equal to that ratio.Its intersection with the lifeline then gives the effective endurance limit for that combination ofσf andσm.Miner’s law for cumulative damageWhen the cyclic load level varies during the fatigue process,a cumulative damage model is often hypothesized.To illustrate,take the lifetime to be N1cycles at a stress levelσ1and N2atσ2. If damage is assumed to accumulate at a constant rate during fatigue and a number of cycles n1 is applied at stressσ1,where n1<N1as shown in Fig.8,then the fraction of lifetime consumedFigure 7:The Goodmandiagram.Figure 8:The concept of fractional lifetime.will be n 1/N 1.To determine how many additional cycles the specimen will survive at stress σ2,an additional fraction of life will be available such that the sum of the two fractions equals one:n 1N 2=1Note that absolute cycles and not log cycles are used here.Solving for the remaining cycles permissible at σ2:n 2=N 2 1−n 1N j =1(1)where n j is the number of cycles applied at a load corresponding to a lifetime of N j .fThe material has been subjected to n 1=105load cycles at a level S =0.6σf ,and we wish to estimate how many cycles n 2the material can now withstand if we raise the load to S =0.7σf .From the S-N relationship,we know the lifetime at S =0.6σf =constant would be N 1=3.98×105and the lifetime at S =0.7σf =constant would be N 2=1.58×104.Now applying Eqn.1:Figure9:Linear S-N curve.n1N2=1×1051.58×104=1n2=1.18×104=A∆K m(2)where da/dN is the fatigue crack growth rate per cycle,∆K=K max−K min is the stress intensity factor range during the cycle,and A and m are parameters that depend the material, environment,frequency,temperature and stress ratio.This is sometimes known as the“Paris law,”and leads to plots similar to that shown in Fig.10.Figure10:The Paris law for fatigue crack growth rates.The exponent m is often near4for metallic systems,which might be rationalized as the damage accumulation being related to the volume V p of the plastic zone:since the volume V p of the zone scales with r2p and r p∝K2I,then da/dn∝∆K4.Some specific values of the constants m and A for various alloys in given in Table1.Table1:Numerical parameters in the Paris equation.mSteel10−11Aluminum10−12Nickel4×10−12Titanium10−11Prob.34.A steel alloy has an S-N curve that falls linearly from 240kpsi at 104cycles to 135kpsi at 106cycles.A specimen is loaded at 160kpsi alternating stress for 105cycles,after which the alternating stress is raised to 180kpsi.How many additional cycles at this higher stress would the specimen be expected to survive?Prob.45.Consider a body,large enough to be considered infinite in lateral dimension,containing a central through-thickness crack initially of length 2a 0and subjected to a cyclic stress of amplitude ∆σ.Using the Paris Law (Eqn.2),show that the number of cycles N f needed for the crack to grow to a length 2a f is given by the relationlna f2m A (∆σ)m πm/2N fe the expression obtained in Prob.5to compute the number of cycles a steel component can sustain before failure,where the initial crack halflength is 0.1mm and the critical crack halflength to cause fracture is 2.5mm.The stress amplitude per cycle is 950MPa.Take the crack to be that of a central crack in an infinite plate.e the expression developed in Prob.5to investigate whether it is better to limit the size a 0of initial flaws or to extend the size a f of the flaw at which fast fracture occurs.Limiting a 0might be done with improved manufacturing or better inspection methods,and increasing a f could be done by selecting a material with greater fracture toughness. For the“baseline”case,take m=3.5,a0=2mm,a f=pute the percentage increase in N f by letting(a)the initialflaw size to be reduced to a0=1mm,and(b) increasing thefinalflaw size to N f=10mm.。
土木工程专业英语(苏小卒版)翻译
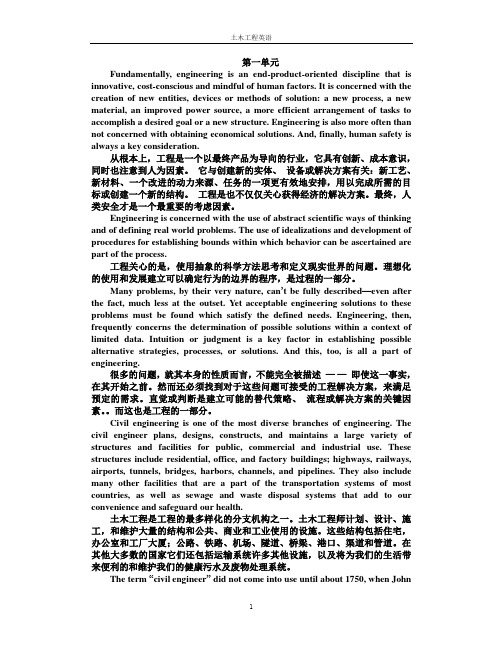
土木工程英语
Smeaton, the builder of famous Eddystone lighthouse near Plymouth, England, is said to have begun calling himself a “civil engineer” to distinguish himself from the military engineers of his time. However, the profession is as old as civilization.
土木工程英语
第一单元 Fundamentally, engineering is an end-product-oriented discipline that is innovative, cost-conscious and mindful of human factors. It is concerned with the creation of new entities, devices or methods of solution: a new process, a new material, an improved power source, a more efficient arrangement of tasks to accomplish a desired goal or a new structure. Engineering is also more often than not concerned with obtaining economical solutions. And, finally, human safety is always a key consideration. 从根本上,工程是一个以最终产品为导向的行业,它具有创新、成本意识, 同时也注意到人为因素。 它与创建新的实体、 设备或解决方案有关:新工艺、 新材料、一个改进的动力来源、任务的一项更有效地安排,用以完成所需的目 标或创建一个新的结构。 工程是也不仅仅关心获得经济的解决方案。最终,人 类安全才是一个最重要的考虑因素。 Engineering is concerned with the use of abstract scientific ways of thinking and of defining real world problems. The use of idealizations and development of procedures for establishing bounds within which behavior can be ascertained are part of the process. 工程关心的是,使用抽象的科学方法思考和定义现实世界的问题。理想化 的使用和发展建立可以确定行为的边界的程序,是过程的一部分。 Many problems, by their very nature, can’t be fully described—even after the fact, much less at the outset. Yet acceptable engineering solutions to these problems must be found which satisfy the defined needs. Engineering, then, frequently concerns the determination of possible solutions within a context of limited data. Intuition or judgment is a key factor in establishing possible alternative strategies, processes, or solutions. And this, too, is all a part of engineering. 很多的问题,就其本身的性质而言,不能完全被描述 — — 即使这一事实, 在其开始之前。然而还必须找到对于这些问题可接受的工程解决方案,来满足 预定的需求。直觉或判断是建立可能的替代策略、 流程或解决方案的关键因 素。。而这也是工程的一部分。 Civil engineering is one of the most diverse branches of engineering. The civil engineer plans, designs, constructs, and maintains a large variety of structures and facilities for public, commercial and industrial use. These structures include residential, office, and factory buildings; highways, railways, airports, tunnels, bridges, harbors, channels, and pipelines. They also include many other facilities that are a part of the transportation systems of most countries, as well as sewage and waste disposal systems that add to our convenience and safeguard our health. 土木工程是工程的最多样化的分支机构之一。土木工程师计划、设计、施 工,和维护大量的结构和公共、商业和工业使用的设施。这些结构包括住宅, 办公室和工厂大厦;公路、铁路、机场、隧道、桥梁、港口、渠道和管道。在 其他大多数的国家它们还包括运输系统许多其他设施,以及将为我们的生活带 来便利的和维护我们的健康污水及废物处理系统。 The term “civil engineer” did not come into use until about 1750, when John
国际知名的力学期刊

国内的话力学杂志有《力学进展》、《力学与实践》等。
给lz一些国际的期刊。
国际知名的力学期刊刊名原文名创刊年附注《应用数学和力学》(中国) (AppliedMa hematics and Mechanics) 1980《应用数学和力学》编辑委员会《热应力杂志》(美)Journal of Thermal Stresses 1978 美国Hemispheres Publishing Co.《国际非线性力学杂志》(英) International Journal of Non-Linear Mechanics 1966 英国Pergamon Press Ltd.《国际固体与结构杂志》International Journal of Solids and Structures 1965 英国Pergamon Press Ltd.《国际多相流杂志》(英)International Journal of Multiphase Flow 1973 英国Pergamon Press Ltd.《地震工程与结构动力学》(英)Earthquake Engineering Structural Dynamics 1972 英国John Wiley Sons Ltd.《国际热与热流杂志》(英) International Journal of Heat and FluidFlow 1979 英国Mechanical Engineering Publi-CationsLtd.《国际地震工程与土壤动力学杂志》(英)International Journal of Earthquake Engineering Soil Dynamics1981 英国CML Publications《工程断裂力学》(英)Engineering Fracture Mechanics 1968 英国Pergamon Press Ltd.《国际压力容器与管道杂志》(英)The International Journal Of Pressure Vessels Piping 1973 英国Applied Science Publishers Ltd.《国际工程数值方法杂志》(英)International Journal for Numerical Methodsin Engineering 1969 英国John Wiley Sons Ltd.《工程材料与结构的疲劳》(英)Fatigue of Engineering Materials and Structures 1978 英国Pergamon Press Ltd《国际疲劳杂志》(英) International Journal of Fatigue 1979 英国IPC Science and Technology Press.《国际岩石力学与采矿学及地质力学文摘》(英)International Journal of Rock Mechanics MiningScienc Geomechanics ABSTRACTS 1964 英国Pergamon Press Ltd.《水利》(法)La Houille Blanche 1902 法国《理论与应用力学杂志》(法)Journal de Mecanique Theorique et Appliquee (Le) 1962 法国Centrale des revues DunodGauthier-Villars《工程师文献》(联邦德国)Ingenieur-Archiv 1929 联邦德国Springer-Verlag 《岩石力学与岩石工程》(奥地利)Rock Mechanics Rock Engineering 1929 奥地利Springer-Verlag《固体力学文献》(荷兰)Solid Mechanics Archives 1976 荷兰Martinus Nijhoff Publishers.《应用力学和工程技术中的计算机方法》(荷兰)Computer Methods in Applied Mechanics and Engineering 1972 荷兰Elsevier Science Publishers.《风工程和工业空气动力学杂志》(荷兰)Journal of Wind Engineering and Industrial Aerodynamics 1975 荷兰Elsevier Scientific Publishing Company(原名为Journal of Industrial Aerodynamics,1980年改为现名)《国际断裂杂志》(荷兰)International Journal of Fracture 1965 荷兰Martinus Nijhoff Publishers《水利学研究杂志》(荷兰)Journal of Hydraulic Research 1963 荷兰International Assiciation for Hydraulic Research《非牛顿流体力学杂志》(荷兰)Journal of Non-Newtonian Flluid Mechanics 1975 荷兰Elsevier Scientific Publishing Company《波动》(荷兰)Wave Motion 1979 荷兰North-Holland Publishing Co.《土木工程学报》(中国)China Civil Engineering 1954 中国土木工程学会China Civil Engineering Society《力学学报》(中国)Acta Me-chanica Subuca 1957 中国力学学会《力学学报》编辑委员会(The Editorial Board of ACTAMECHANIC A SINICA,the Chinese Society of Theoretical and Applied Mechanics)《力学译丛》(中国)1964 中国科学技术情报研究所分所《力学进展》(中国)1982 中国科学院力学研究所《应用力学》(中国)1982 中国科学技术情报研究所分所《固体力学学报》(中国)Acta Mechanica Solida Sinica 1980 《固体力学》学报编辑委员会员《应用数学和力学》(中国)Applied Mathematics and Mechanics 1980 《应用数学和力学》编辑委员会《建筑结构学报》(中国)Jour-nal of Building Structures 1980 中国建筑学会《上海力学》(中国)1980 《上海力学》编辑部《爆炸与冲击》(中国)1981 《爆炸与冲击》编辑部《振动与冲击》(中国)1982 《振动与冲击》编辑委员会《空气动力学学报》(中国)Acta Aerodynamica Sinica 1983 《空气动力学学报》编辑委员会《数学物理学报》(中国)1981 《数学物理学报》编辑委员会《实验应力分析学会会报》(美)Proceedings of the Society for Experimental StressAnalysis 1943 美国实验应力分析学会(Society for Experimental Stress Analysis)《实验力学》(美)Experimental Mechanics 1961 美国实验应力分析学会(Society for Experimental Stress Analysis)《结构力学杂志》(美)Journal of Structural Mechanics 1972 美国Marcel Dekker Ine.《流变学杂志》(美)Journal of Rheology 1957 美国John Wiley Sons Inc. Publishers.《液压与气体力学》(美)Hydraulics Pneumatics; Magazine of Fluid Power and Control Systems 1948 美国Penton/IPC《流体物理学》(美)Physics of Fluids 1958 美国物理学会(American Institute of Physics)《流体力学年评》(美)Annual Review of Fluid Mechanics 1969 美国Annual Review Inc.《应用力学杂志》(美)Journal of AppliedMechanics 1935 美国机械工程师学会(American Society ofMechanical Engineers)《实验应力分析学会年度春季会议录》(美)Proceedingsof the SESA Annual Spring Meeting 美国实验应力分析学会(Society for Experimental Stress Analysis)《聚合物科学杂志》(美)Journal of Polymer Science 1946 美国John Wiley Sons Inc Publishers《生物工程学杂志》(美)Journal of BiomechanicalEngineering 1977 美国机械工程师学会(American Society ofMechanical Engineers)《复合材料杂志》(美)Journal of Composite Materials 1967 美国Technomic Publishing Company Inc.《流体工程学杂志》(美)Journal of FluidsEngineering 1973 美国机械工程师学会(American Society ofMechanical Engineers)《美国土木工程师学会会报--工程力学组杂志》(美)Proceedings of the American Society of CivilEngineers- Journal of the Engineer Mechanics Division 1873 美国机械工程师学会(American Society of Civil Engineers)《自动车工程师学会汇刊》(美)SAE Transactions 1906 自动车工程师学会(Society of Automotive Engineers)《船舶研究杂志》(美)Journal of ShipResearch 1893 造船与轮机工程师协会(Society of NavalArchitects Marine Engineers)《美国航空与航天学会志》(美)AIAA Journal 1930 美国航空与航天学会(American Institute of Aeronautics Astronautics)《苏联流体力学研究》(美)Fluid Mechanics-Soviet Research 1972 美国Scripta Publishing Co.《流体动力学》(美)Fluid Dynamics 1966 美国Plenum Publishing Co.《伦敦皇家学会会报,A辑:数学及物理科学》(英)Proceedings of the Royal Society of London,A:Mathematical Physical Sciences 1854 英国皇家学会(The Royal Society of London)《伦敦皇家学会哲学汇刊,A 辑数学与物理科学》(英)Philosophical Transactions of the RoyalSociety of London,SeriesA:Mathematical PhysicalSciences 1854 英国皇家学会(The Royal Society of London)1887年(第178卷)起分A,B两辑出版《力学研究通讯》(英)Mechanics Research Communications 1974 英国Pergamon Press Ltd《生物流变学》(英)Biorheology 1963 英国Pergamon Press Ltd.《生物力学杂志》(英)Journal of Biomechanics 1968 英国Pergamon Press Ltd.《材料科学杂志》(英)Journal of Materials Science 1966 英国Chapman and Hall Ltd.《应变》(英)Strain 1964 英国应变测量学会(British Society for Strain Measurement)《工程设计应变分析杂志》(英)Journal of Strain Analysis for Engineering Design 1965 英国Mechanical EngineeringPublications Ltd.《力学研究》(英)Research Mechanica 1980 英国Applied Science Publishers《计算机与结构》(英)Computers Structures 1971 英国《计算机与流体》(英)Computers Fluid 1971 英国Pergamon Press Ltd. 《水力气体机械动力》(英)Hydraulic Pneumatic Mechanical Power 1955 英国Trade Technical Press Ltd. Ltd.《飞机工程》(英)Aircraft Engineering 1929 英国Bunhill Publications Ltd. 《航空季刊》(英)Aeronautical Quarterly 1949 英国皇家学会(Royal Aeronautical Society)《航空杂志》(英)Aeronautical Journal 1897 英国皇家学会(Royal Aeronautical Society)《星际航行学报》(英)ActaAstronautica 1955 英国1974年改为现名,1955~1973年刊名为Astronautica Acta,Pergamon Press Ltc.《应用数学与力学杂志》(英)Journal of Applied Mathematics Mechanics 1958 英国1974年改为现名,1955~ 1973年刊名为AstronauticaActa,Pergamon Press Ltd《理性力学与分析文献》(联邦德国)Archive for Rational Mechanics and Analysis 1957 联邦德国springer-Verlag《流变学学报》(联邦德国)Rheologica Acta 1958 联邦德国Dr. Dietrich Steinkopff Verlag《流体力学实验》(联邦德国)Experiments in Fluid 1983 联邦德国springer-Verlag《油压力学与气体力学》(联邦德国)Olhydraulik und Pneumatik 1957 联邦德国Krausskopf Verlagsgruppe《数学生物学杂志》(联邦德国)Journal of Mathematical Biology 1974 联邦德国springer-Verlag《热力学与流体力学》(联邦德国)Warme-und Stoffubertragung 1968 联邦德国springer-Verlag《法国流变学小组手册》《通报》(法)Cahiers et Bulletin du Groupe Franais de rheologie 1965 法国《法国科学院会议周报,A-B辑:数理科学》(法)Comptes Rendus Hebdomadaires des Seances deL’Academie des Sciences, Series A et B:”Sciences Mathematiques,Sciences Physiques” 1835 法国Centrale des Revues Dunod Gauthier-Villars《应用力学纪事》(法)Journal de Mecanique Appliquee 1977 法国Centrale des Revues Dunod Gauthier-Villars《力学》(意)Mechanica 1966 意大利Pitagora Editrice《力学学报》(奥地利)Acta Mechanica 1965 奥地利Springer-Verlag《弹性体杂志》(荷)Journal of Elasticity 1971 荷兰artinus Nijhoff Publishers《天体力学》(荷)Celestial Mechanics 1969 荷兰Publishing Co.《工程数学杂志》(荷)Journal of Engineering Mathematics 1966 荷兰Martinus Nijhoff Publishers《材料力学》(荷)Mechanics of Materials 1981 荷兰North-Holland Publishing Co.《澳大利亚地质力学杂志》(澳)The Australian Geomechanics Journal 1971 澳大利亚《加拿大航空与空间杂志》(加)Canadian Aeronautics SpaceJournal 1955 加拿大,1962年改为现名,1955~1961年刊名为:Canadian Aeronautics Journal.《核工程与设计》(瑞士)Nuclear Engineering and Design 1965 瑞士Elsevier Sequoia .《应用数学与力学杂志》(民主德国)ZAMM-Zeitschrift fur Angewandt Mathematikund Mechanik 1921 民主德国Akademic-Verlag《理论与应用力学》(波兰)Mechanika Teoretyczna i Stosowana 1964 波兰PWN《工程汇刊》(波兰)Rozprawy Inzynierskie 1953 波兰PWN《力学文献集》(波兰)Archives of Mechanics 1849 波兰PWN《罗马尼亚技术科学杂志, 应用力学辑》(罗)Revue Roumaine des sciences Techniques,Serie Mecanique Appliquee1956 罗马尼亚科学出版社《应用力学研究》(罗)Studii si Cerctari de Mecanica Applicata 1942 罗马尼亚科学出版社《日本应用力学全国会议录》(日)Proceedings of the Japan National Congress of Applied Mechanics 1953 日本中央科学社《材料》(日)Journal of the Society of Materials Science 1952 日本材料学会《日本机械学会论文集》(日)Transactions of the Japan Society of Mechanical Engineer 1935 日本机械学会《土木协会论文报告集》(日)Proceedings of the Japan Society of Civil Engineers 1944 日本土木工学会《日本造船协会志》(日)Bulletin of the society of Naval Archiects of Japan 1915 日本造船协会《流体工程学》(日)流体工学1965 日本产业开发社(原名:学,1965~)《日本材料强度学会志》日本材料强度学会志1967 日本材料强度学会《力学研究所报告》(日)力学研究所报告1967 日本力学研究所《日本流变学会志》(日)日本一学会志1973 日本流变学会《应用数学与力学》(苏联)1936 苏联《苏联科学院通报:固体力学》(苏)1966 苏联,美国出版有英译本《磁流体力学》(苏)1965 苏联,美国出版有英译本《燃烧与爆炸物理学》(苏)1965 苏联《应用力学与物理学杂志》(苏)1960 苏联,美国出版有英译本《应用力学》(苏)1955 苏联《复合材料力学》(苏)1965 苏联《建筑力学与建筑物计算》(苏)1959 苏联《莫斯科大学力学通报》(美)Moscow University Mechanics Bulletin 1969 美国Allerton Press Inc (译自俄文)《得克萨斯大学巴尔科研究中心年报》(美)Annual Repoet-Balcones Research Center, Texas at Austin 美国《剑桥哲学会数学汇刊》(英)Re Mathematical Proceedings of the Cambridge Philosophical Society 1977 英国Cambridge ,1977年改为现名,1843~1976 年名为Proceedings of Cambridge Philosophical Society;Mathematical Physical Sciences《力学与应用数学季刊》(英)Quarterly Journal of Mechanics and Applied Mathematics 1948 英国《流体力学杂志》(英)Journal of Fluid Mechanics 1956 英国Cambridge 《应用力学研究所报告》(日)Reports of Research Institute for Applied Mathematics 1952 日本九州大学应用力学研究所《东京大学航天研究所报告》ISAS (Institute of Space Aeronautical Science,Univ. Tokyo) 日本东京大学航天研究所《布加勒斯特乔治乌德治工学院通报:力学辑》(罗)Buletinul Institutului Politehnic“Gheorghe Gheorghiu-Dij” 1949 罗马尼亚《列宁格勒大学通报:数学,力学和天文学类》(苏)1946 苏联《莫斯科大学通报:数学力学类》(苏)1946 苏联《国外科技资料馆藏目录━数学,力学》(中国) 中国科学技术情报研究所《力学文摘━流体力学部分》(中国)1958 中国科学技术情报研究所重庆分所翻译,苏联科学院科学情报研究所文摘编辑委员会编辑《力学文摘━一般力学部分》(中国)1958 中国科学技术情报研究所重庆分所翻译,苏联科学院科学情报研究所文摘编辑委员会编辑《力学文摘━弹性力学部分》(中国)1958 中国科学技术情报研究所重庆分所翻译,苏联科学院科学情报研究所文摘编辑委员会编辑《数学文摘》(美)Mathematical Reviewswith Index toMathematical Reviews 1940 美国数学会American Mathematical Society《冲击与振动研究辑要》(美)Shock and Vibration Digest 1969 美国冲击与振动情报中心《流变学通报》(美)Rheology Bulletin 1937 美国物理学会American Institute ofPhysics《应用力学文摘》(美)Applied Mechanics Reviews 1948 美国机械工程师协会American Society of Mechanical Engineers《地震工程文摘杂志》(美)Abstracts Journal in Earthquake Engineering 1968 美国加利福尼亚大学伯克利分校地震工程研究中心Univ. of California, Berkeley, Earthquake Engineering Research Center《工程索引》(美)Engineering Index (Annual) 1884 美国Engineering Index Inc.《美国土木工程师学会汇刊》(美)Transactions of the American Society of Civil百度文库- 好好学习,天天向上Engineering 1852 美国土木工程师学会American Society of Civil Engineering 《科学引文索引》(美)Science Citation Index 1961 美国科学情报研究所Institute of Scientific Information《土木工程水利文摘》(英)Civil Engineering HydraulicsAbstracts 1968 英国流体力学研究协会British Hydromechanics Research Association《流变学文摘》(英)Rheology Abstracts 1940 英国Pergamon Press 《固体-液体流文摘》(英)Solid-Liquid FlowAbstracts 1973 英国流体力学研究协会British HydromechanicsResearch Association《工业空气动力学文摘》(英)Industrial Aerodynamics Abstracts 1970 英国流体力学研究协会British Hydromechanics《流体动力学文摘》(英)Fluid Power Abstracts 1965 英国流体力学研究协会Hydromechanics Research Association《英国土木工程师协会文摘》(英)ICE Abstracts 1972 英国流体力学研究协会,1974 年改为现名(The Institution of Civil Engineers)《法国全国科学研究中心文摘通报,第130辑:数学,物理,光学,声学,力学,热学》(法)Bulletin Signaletique du 130 hysique Mathematique, Optique, Acoustique, Mecanique, Chaleur 1961 法国全国科学研究中心《科学技术文献速报:机械工学编》(日)Currdnt Bibliography on Science Technology 1975 日本科学技术情报中心(日本科学技术情报)《文摘杂志:力学(综合本)》(苏)1953 苏联全苏科学技术情报研究所《力学与实践》(中国)1979 《力学与实践》编辑委员会《美国物理学杂志》(美)American Journal of Physics 1933 美国物理学会American Institute-11。
最新汽车专业英语原文及翻译
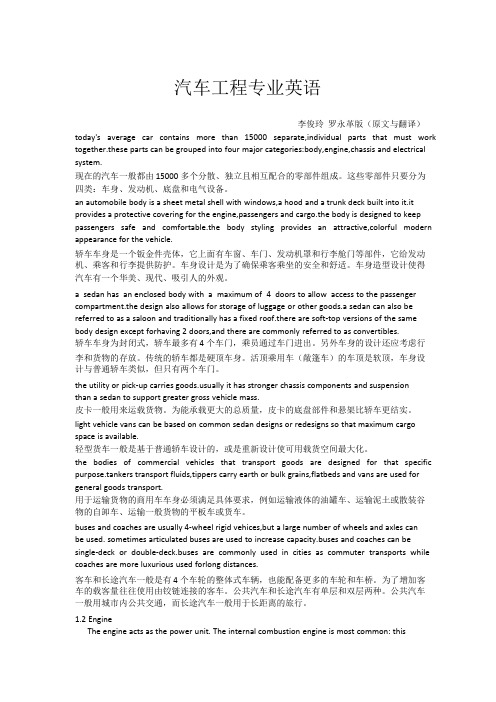
obtains its power by burning a liquid fuel inside the engine cylinder. There are two types of engine: gasoline (also called a spark-ignition engine) and diesel (also called a compression-ignition engine). Both engines are called heat engines; the burning fuel generates heat which causes the gas inside the cylinder to increase its pressure and supply power to rotate a shaft connected to the power train. 发动机作为动力设备,常见的类型是内燃机,其原理是通过发动机缸内的液体燃料燃烧而产 生能量。发动机可分为两类:汽油机(点燃式)和柴油机(压燃式),都属于热力发动机。 燃料燃烧产生热量使缸内气体气压上升,从而产生能量,驱动与动力传动系相连接的轴旋转。 The way engine cylinders are arranged is called engine configuration. In-line engines have the cylinders in a line. This design creates a simply cast engine block. In vehicle applications, the number of cylinders is normally from 2 up to 6. Usually, the cylinders are vertical. As the number of the cylinders increase, the length of the block and crankshaft can become a problem. One way to avoid this is with a V configuration. This design makes the engines block and crankshaft shorter and more rigid. 发动机的布置即发动机气缸的排列方式。 发动机缸体按直线排列的即直列式,这种布置使 得发动机缸体结构简单。汽车发动机一般为 2~6 缸,通常气缸是垂直放置的,但气缸数量的 增加会导致缸体和曲轴的长度过大,解决问题的措施之一就是采用 V 型布置,这种布置方 式可以使发动机缸体和曲轴长度尺寸更短,从而大大增加刚度。 An engine located at the front can be mounted longitudinally and can drive either the front or the rear wheels. Rear engine vehicles have the engine mounted behind the rear wheels. The engine can be transverse or longitudinal and usually drives the rear wheels only. 前置发动机可以纵向布置,能够驱动前轮或后轮。后置发动机布置在后轮后侧,发动机即可 纵向布置又可横向布置,一般只能驱动后轮。 1.3 chassis 底盘 the chassis is an assembly of those systems that are the major operating parts of a vehicle.the chassis includes the power train,steering,suspension,and braking systems. 底盘由汽车的主要操作系统组装而成。包括传动系、行驶系、转向系和制动系四部分。 1)Powertrain system conveys the drive to the wheels.传动系-将驱动力传递到车轮。 2)Steering system controls the direction of movement.转向系-控制汽车的行驶方向。 3)Suspension and wheels absorbs the road shocks.悬挂系-吸收路面震动 4)Brake slows down the vehicle.制动系-使汽车减速缓行。 1.3.1 power train system 动力传动系统 The power train transfers turning effort from the engine to the driving wheels.a power train ca n include a clutch manual transmission or a torque converter for automatic transmission, a dr ive shaft, final drive and differential gears and driving axles. Alternatively,a transaxle may be use d. A transaxle is a self-contained unit with a transmission, final drive gears and differential locate d in one casing. 传动系把发动机输出的扭矩传递给驱动轮。传动系包括离合器(对应机械变速器)或液力变 矩器(对应液力自动变速器)、变速器、驱动轴、主减速器、差速器和驱动桥。另外有些传 动系采用由变速器、主减速器和差速器组成的一体式的变速驱动桥。(或者采用一个独立的 变速驱动桥,即变速器……在同一个箱体内。) A vehicle with a manual transmission uses a clutch to engage and disengage the engine from the power train. Engine torque is transmitted through the clutch to the transmission or transaxle.the transmission contains sets of gears that increase or decrease the torque before it is transmitted
工作原理 英文
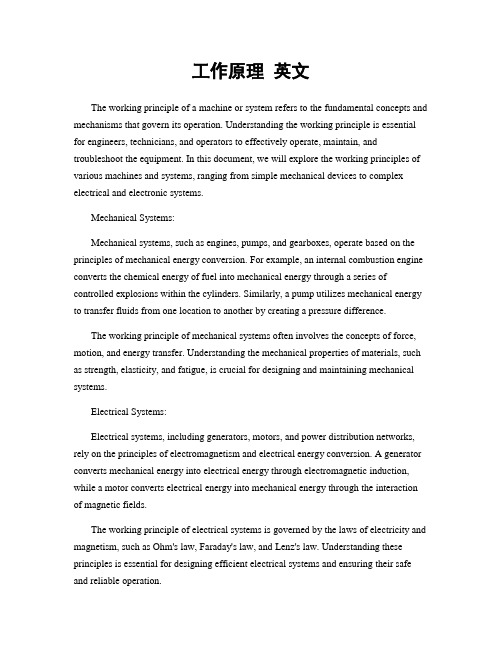
工作原理英文The working principle of a machine or system refers to the fundamental concepts and mechanisms that govern its operation. Understanding the working principle is essential for engineers, technicians, and operators to effectively operate, maintain, and troubleshoot the equipment. In this document, we will explore the working principles of various machines and systems, ranging from simple mechanical devices to complex electrical and electronic systems.Mechanical Systems:Mechanical systems, such as engines, pumps, and gearboxes, operate based on the principles of mechanical energy conversion. For example, an internal combustion engine converts the chemical energy of fuel into mechanical energy through a series of controlled explosions within the cylinders. Similarly, a pump utilizes mechanical energy to transfer fluids from one location to another by creating a pressure difference.The working principle of mechanical systems often involves the concepts of force, motion, and energy transfer. Understanding the mechanical properties of materials, such as strength, elasticity, and fatigue, is crucial for designing and maintaining mechanical systems.Electrical Systems:Electrical systems, including generators, motors, and power distribution networks, rely on the principles of electromagnetism and electrical energy conversion. A generator converts mechanical energy into electrical energy through electromagnetic induction, while a motor converts electrical energy into mechanical energy through the interaction of magnetic fields.The working principle of electrical systems is governed by the laws of electricity and magnetism, such as Ohm's law, Faraday's law, and Lenz's law. Understanding these principles is essential for designing efficient electrical systems and ensuring their safe and reliable operation.Electronic Systems:Electronic systems, such as computer circuits, communication devices, and control systems, operate based on the principles of electronic signal processing and information transmission. For example, a microprocessor processes digital signals to perform arithmetic and logic operations, while a communication device modulates and demodulates analog signals to transmit and receive information.The working principle of electronic systems involves the manipulation of electronic signals using components such as transistors, diodes, and integrated circuits. Understanding the behavior of these components is crucial for designing and troubleshooting electronic systems.Overall, the working principle of machines and systems encompasses a wide range of physical and engineering principles, including mechanics, thermodynamics, electromagnetism, and information theory. By understanding these principles, engineers and technicians can effectively design, operate, and maintain various machines and systems to meet the needs of modern society.。
Engineering Ethics
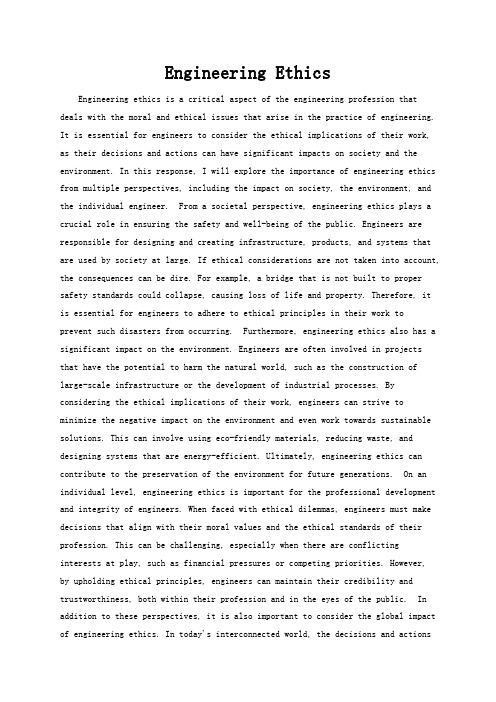
Engineering EthicsEngineering ethics is a critical aspect of the engineering profession that deals with the moral and ethical issues that arise in the practice of engineering. It is essential for engineers to consider the ethical implications of their work, as their decisions and actions can have significant impacts on society and the environment. In this response, I will explore the importance of engineering ethics from multiple perspectives, including the impact on society, the environment, and the individual engineer. From a societal perspective, engineering ethics plays a crucial role in ensuring the safety and well-being of the public. Engineers are responsible for designing and creating infrastructure, products, and systems that are used by society at large. If ethical considerations are not taken into account, the consequences can be dire. For example, a bridge that is not built to proper safety standards could collapse, causing loss of life and property. Therefore, itis essential for engineers to adhere to ethical principles in their work toprevent such disasters from occurring. Furthermore, engineering ethics also has a significant impact on the environment. Engineers are often involved in projectsthat have the potential to harm the natural world, such as the construction of large-scale infrastructure or the development of industrial processes. By considering the ethical implications of their work, engineers can strive to minimize the negative impact on the environment and even work towards sustainable solutions. This can involve using eco-friendly materials, reducing waste, and designing systems that are energy-efficient. Ultimately, engineering ethics can contribute to the preservation of the environment for future generations. On an individual level, engineering ethics is important for the professional development and integrity of engineers. When faced with ethical dilemmas, engineers must make decisions that align with their moral values and the ethical standards of their profession. This can be challenging, especially when there are conflictinginterests at play, such as financial pressures or competing priorities. However,by upholding ethical principles, engineers can maintain their credibility and trustworthiness, both within their profession and in the eyes of the public. In addition to these perspectives, it is also important to consider the global impact of engineering ethics. In today's interconnected world, the decisions and actionsof engineers can have far-reaching consequences beyond their immediate surroundings. For example, the design of a new technology in one part of the world can affect communities and ecosystems in distant regions. Therefore, it is crucial for engineers to take a global perspective when considering the ethicalimplications of their work, and to consider the potential impact on a broader scale. In conclusion, engineering ethics is a fundamental aspect of the engineering profession that must be considered from multiple perspectives. Fromthe societal impact to the environmental consequences, and the individualengineer's professional integrity, ethical considerations are essential inensuring that engineering serves the greater good. By upholding ethical principles, engineers can contribute to a safer, more sustainable, and more equitable worldfor all.。
international journal of fatigue简介 -回复
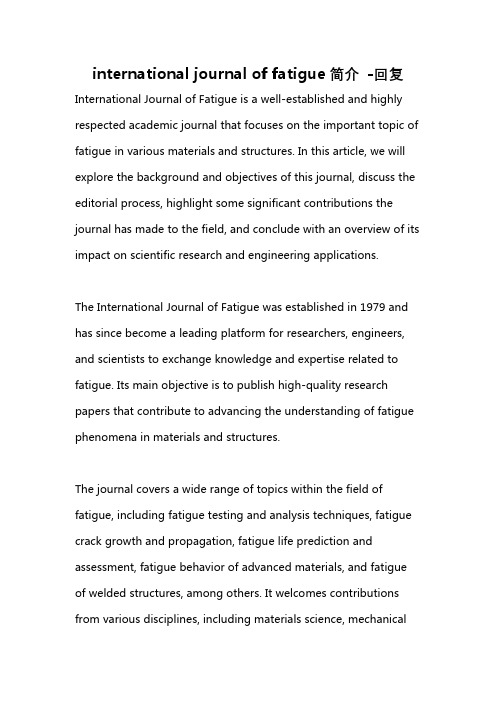
international journal of fatigue简介-回复International Journal of Fatigue is a well-established and highly respected academic journal that focuses on the important topic of fatigue in various materials and structures. In this article, we will explore the background and objectives of this journal, discuss the editorial process, highlight some significant contributions the journal has made to the field, and conclude with an overview of its impact on scientific research and engineering applications.The International Journal of Fatigue was established in 1979 and has since become a leading platform for researchers, engineers, and scientists to exchange knowledge and expertise related to fatigue. Its main objective is to publish high-quality research papers that contribute to advancing the understanding of fatigue phenomena in materials and structures.The journal covers a wide range of topics within the field of fatigue, including fatigue testing and analysis techniques, fatigue crack growth and propagation, fatigue life prediction and assessment, fatigue behavior of advanced materials, and fatigue of welded structures, among others. It welcomes contributions from various disciplines, including materials science, mechanicalengineering, civil engineering, aerospace engineering, and metallurgy.The editorial process of the International Journal of Fatigue is rigorous and ensures the publication of only the highest-quality research. Upon submission, each manuscript undergoes a thorough peer-review process by experts in the field. The reviewers assess the novelty, the scientific rigor, and the significance of the findings presented in the manuscript. Based on the reviewers' recommendations, the editor-in-chief makes a final decision regarding acceptance, revision, or rejection of the submission. This stringent review process ensures that only valuable and reliable research is published in the journal.Over the years, the International Journal of Fatigue has made significant contributions to the field. It has published numerous seminal studies that have expanded our understanding of fatigue phenomena and influenced the development of fatigue design codes and guidelines. For instance, the journal has featured papers on novel fatigue testing methodologies, such as ultrasonic fatigue testing and full-scale structural testing, which have provided valuable insights into the fatigue behavior of variousmaterials and structures. Additionally, the journal has published research on innovative fatigue life prediction models, which have enhanced the accuracy of fatigue life assessment and contributed to the development of more reliable designs.The impact of the International Journal of Fatigue extends beyond academia. The research published in the journal has contributed to advancements in engineering practice and has influenced the design of critical structures and components, such as aircraft, bridges, and offshore structures. The findings presented in the journal have also led to improvements in manufacturing processes, material selection, and maintenance strategies, ultimately enhancing the reliability and safety of various engineering systems.In conclusion, the International Journal of Fatigue is a highly esteemed academic journal that plays a crucial role in advancing the understanding of fatigue phenomena in various materials and structures. Through its rigorous editorial process and publication of high-quality research, the journal has made significant contributions to the field. Its findings have impacted bothacademic research and engineering applications, ultimately improving the reliability and safety of structures in multiple industries.。
交付工程师英语
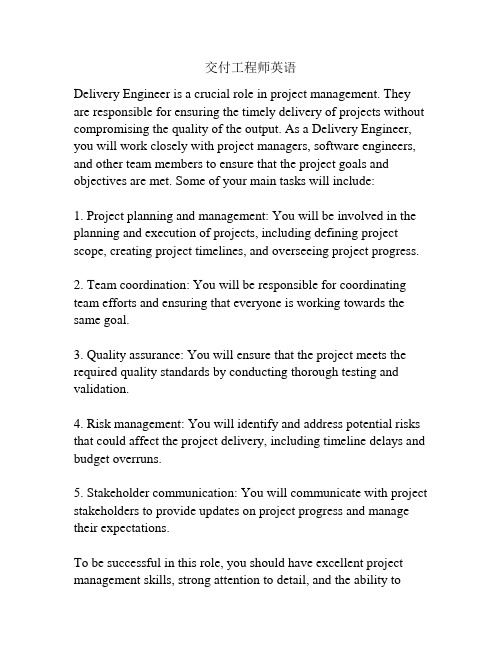
交付工程师英语Delivery Engineer is a crucial role in project management. They are responsible for ensuring the timely delivery of projects without compromising the quality of the output. As a Delivery Engineer, you will work closely with project managers, software engineers, and other team members to ensure that the project goals and objectives are met. Some of your main tasks will include:1. Project planning and management: You will be involved in the planning and execution of projects, including defining project scope, creating project timelines, and overseeing project progress.2. Team coordination: You will be responsible for coordinating team efforts and ensuring that everyone is working towards the same goal.3. Quality assurance: You will ensure that the project meets the required quality standards by conducting thorough testing and validation.4. Risk management: You will identify and address potential risks that could affect the project delivery, including timeline delays and budget overruns.5. Stakeholder communication: You will communicate with project stakeholders to provide updates on project progress and manage their expectations.To be successful in this role, you should have excellent project management skills, strong attention to detail, and the ability towork well under pressure. You should also possess excellent communication and interpersonal skills to work effectively with other team members and stakeholders. Experience in software development and familiarity with Agile methodologies are key requirements for most Delivery Engineer positions.。
- 1、下载文档前请自行甄别文档内容的完整性,平台不提供额外的编辑、内容补充、找答案等附加服务。
- 2、"仅部分预览"的文档,不可在线预览部分如存在完整性等问题,可反馈申请退款(可完整预览的文档不适用该条件!)。
- 3、如文档侵犯您的权益,请联系客服反馈,我们会尽快为您处理(人工客服工作时间:9:00-18:30)。
Objective
• Overview nature/consequences of the fatigue failure mechanism • Determine number of cycles required to
– develop a fatigue crack – propagate a fatigue crack
Southern Regional Office 1950 Stemmons Freeway - Suite 5068 Dallas, TX 75207-3109 214-800-4900 800-445-2388 214-800-4902 (FAX) Western Regional Office 119-C Paul Drive San Rafael, CA 94903-2022 415-499-1148 800-624-9002 415-499-1338 (FAX)
• Bend wire repeatedly until fracture
• Note:
– life (number of applied load cycles) depends on:
• applied stress amplitude • component “quality” (notches, scratches, etc.)
9
Exercise
Estimate the fatigue lifetime needed for:
– Automobile axle – Railroad rail – Commercial aircraft components
• landing gear • lower wing skin
– Highway drawbridge mechanism – Space shuttle solid propellant rocket motor cases
Crack Length (a) Fracture
Stress
Crack a Time
Crack Nucleation Crack Growth
Elapsed Cycles N
• Fatigue life depends on initial quality, load, . . .
5
Paper Clip Experiment
14
Model Stress-life (S-N) Curve
Log ∆S/2
• Se = endurance limit
for steels – Se ~ 0.5 ultimate stress Sult – Se ~ 100 ksi if Sult 〈 200 ksi
∆S/2 = σf ’ (2N)b
• Control ∆ε • Measure
– “Reversals” (2Nf) to failure (1 cycle = 2 reversals) – Stable stress range ∆σ needed to maintain ∆ε
18
∆ε
∆ε time
σ ∆σ
Note: “stable” ∆σ usually occurs by mid-life (2Nf /2)
– What is the required lifetime? – What are consequences of failure?
11
Fatigue Crack Formation
12
Fatigue Crack Formation
Objective
– Characterize resistance to fatigue crack formation – Predict number of cycles to “initiate” small* fatigue crack in component
You can also find information on these courses and all of ASME, including ASME Professional Development, the Vice President of Professional Development, and other contacts at the ASME Website: http://www.asme http://www. .org
– limited by local plastic deformation – strain-life approach better for LCF (Low Cycle Fatigue)
17
Strain-life (ε - N) Approach
Concept: Strain range ∆ε controls life ∆σ Experiment ε
Plastic
• • • • •
4
Buckling Fracture Creep Corrosion Fatigue
Yield Permanent displacement
Displacement
Fatigue Failure Mechanism
• Caused by repeated (cyclic) loading • Involves crack foห้องสมุดไป่ตู้mation, growth, and final fracture
10
Exercise
• Give an example of a High Cycle Fatigue (HCF) application.
– What is the required lifetime? – What are consequences of failure?
• Given an example of a Low Cycle Fatigue (LCF) application.
– heat emitted >> plastic deformation
6
Characteristics of Fatigue
• “Brittle” fracture surface appearance • Cracks often form at free surface • Macro/micro “beach marks”/ “striations”
13
Stress-life (S-N) Approach
Concept: Stress range controls fatigue life
S
S
∆S
∆S/2
S
time
Log cycles N
Note: • Life increases as load amplitude decreases
• Considerable scatter in data • “Run-outs” suggest “infinite life” possible • Life N usually total cycles to failure
15
S-N Curve: Mean Stress
Mean stress effects life
stress ratio R = Smin / Smax Smean = 0.5(Smin + Smax) Sa = 0.5(Smax - Smin) = ∆S/2 S Smax ∆S = 2Sa Smin time
Mean stress models
Sa/Se + Sm /Sult = 1
∆S/2 = (σf ’ - Smean) (2N)b
Stress Amplitude
“Haigh” constant life diagram N = 103 N = 106
Mean Stress
16
S-N Curve: Other Factors
• Discuss implications of fatigue on design and maintenance operations
3
Structural Failure Modes
Force
• Excessive Deformation
– –
Elastic
Force
displacement
time
Cyclic Stress-Strain Curve
Relate stable cyclic stress and strain ranges
∆σ
∆ε
ε
∆ε
σ
σ
∆σ
∆ε time
time
ε
∆σ
Cyclic stress-strain curve ∆σ/2 ∆ε/2
“Hystersis” loop E = elastic modulus K’ = cyclic strength coefficient n’ = strain hardening exponent
Se Log reversals 2N
• σf ’ = fatigue strength coefficient • b = fatigue strength exponent
typically -0.12 < b < -0.05
Note: Measure life in terms of reversals 2N (1 cycle = 2 reversals)
• S-N curves are very sensitive to
– surface finish, coatings, notches – prior loading, residual stresses – specimen size effects, etc.
• Many empirical “knock-down” factors • S-N approach best suited for HCF (High Cycle Fatigue) applications