Cloning_of_artificial_microRNAs
基因工程在克隆方面的英文作文
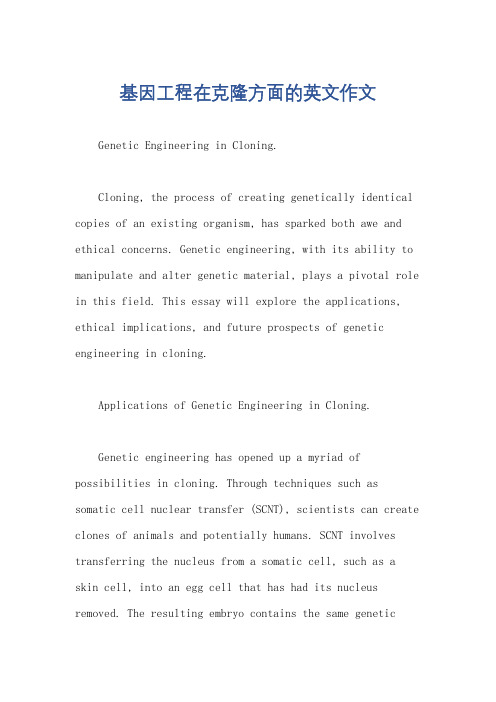
基因工程在克隆方面的英文作文Genetic Engineering in Cloning.Cloning, the process of creating genetically identical copies of an existing organism, has sparked both awe and ethical concerns. Genetic engineering, with its ability to manipulate and alter genetic material, plays a pivotal role in this field. This essay will explore the applications, ethical implications, and future prospects of genetic engineering in cloning.Applications of Genetic Engineering in Cloning.Genetic engineering has opened up a myriad of possibilities in cloning. Through techniques such as somatic cell nuclear transfer (SCNT), scientists can create clones of animals and potentially humans. SCNT involves transferring the nucleus from a somatic cell, such as a skin cell, into an egg cell that has had its nucleus removed. The resulting embryo contains the same geneticmaterial as the somatic cell donor, leading to the development of a genetic clone.Cloning holds great promise in various fields. In medicine, it offers the potential to generate patient-specific stem cells for regenerative therapies, organ transplantation, and disease modeling. By creating genetically identical copies of a patient's cells, scientists can differentiate them into specific cell types needed for transplantation or research. Additionally, cloning can aid in conservation efforts by preserving endangered species and reintroducing them into theirnatural habitats.Ethical Implications of Genetic Engineering in Cloning.While the potential benefits of cloning are undeniable, ethical concerns cannot be overlooked. One primary concern is the potential for reproductive cloning, which would create genetically identical human beings. This raises questions about personal identity, autonomy, and the potential for genetic manipulation for eugenic purposes.Another ethical consideration is the welfare of cloned animals. The process of cloning can be demanding and may result in developmental abnormalities or health problems. Cloning animals for commercial purposes, such as food production or medical research, can raise concerns about animal rights and the ethical treatment of living beings.Future Prospects of Genetic Engineering in Cloning.The future of genetic engineering in cloning holds both challenges and opportunities. Advances in gene editing technologies, such as CRISPR-Cas9, offer greater precision and control in altering genetic material. This could lead to the development of more efficient and targeted cloning techniques.However, ethical concerns and societal debates will continue to shape the future of cloning. International regulations, public engagement, and ethical guidelines are essential to ensure the responsible and ethical use of genetic engineering in this field.Conclusion.Genetic engineering has revolutionized the field of cloning, enabling the creation of genetically identical organisms with potential applications in medicine, conservation, and agriculture. While ethical considerations and societal debates are crucial, the continued responsible and ethical use of genetic engineering in cloning can harness its potential for scientific advancement and societal benefits. As the field progresses, it is imperative that scientific research, ethical dialogue, and public engagement work in tandem to shape a future where cloning is used for the betterment of society without compromising ethical principles.。
克隆的 英语作文
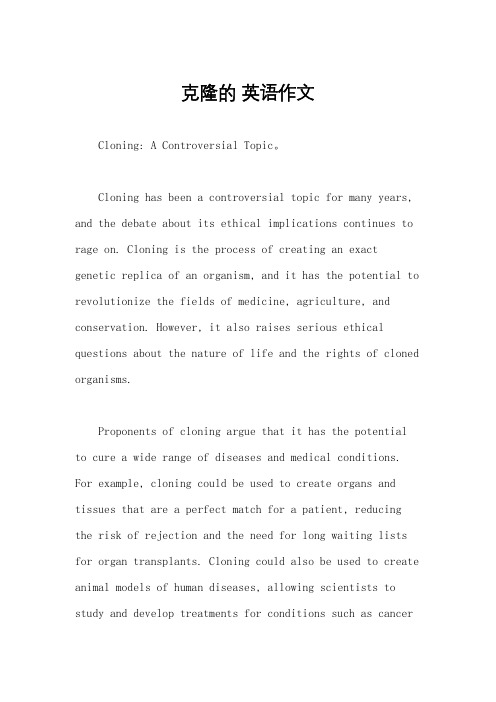
克隆的英语作文Cloning: A Controversial Topic。
Cloning has been a controversial topic for many years, and the debate about its ethical implications continues to rage on. Cloning is the process of creating an exact genetic replica of an organism, and it has the potential to revolutionize the fields of medicine, agriculture, and conservation. However, it also raises serious ethical questions about the nature of life and the rights of cloned organisms.Proponents of cloning argue that it has the potential to cure a wide range of diseases and medical conditions. For example, cloning could be used to create organs and tissues that are a perfect match for a patient, reducing the risk of rejection and the need for long waiting lists for organ transplants. Cloning could also be used to create animal models of human diseases, allowing scientists to study and develop treatments for conditions such as cancerand Alzheimer's disease. In addition, cloning could be used to preserve endangered species by creating genetically identical individuals that could be reintroduced into the wild.On the other hand, opponents of cloning argue that itis unethical to create and manipulate life in this way. They argue that cloning raises serious questions about the nature of individuality and the rights of cloned organisms. Cloned animals may suffer from health problems and genetic abnormalities, and there are concerns about the welfare of cloned animals and the potential for exploitation. In addition, there are concerns about the potential for misuse of cloning technology, such as the creation of designer babies or the cloning of humans for organ harvesting.Despite these concerns, cloning continues to be a topic of interest and research in the scientific community. In recent years, researchers have made significant advances in the field of cloning, including the successful cloning of animals such as sheep, cows, and dogs. These advances have raised hopes for the potential of cloning in fields such asmedicine, agriculture, and conservation.In conclusion, cloning is a complex and controversial topic that raises serious ethical questions about the nature of life and the rights of cloned organisms. While cloning has the potential to revolutionize the fields of medicine, agriculture, and conservation, it also raises serious concerns about the welfare of cloned animals and the potential for misuse of cloning technology. As the field of cloning continues to advance, it is important for society to carefully consider the ethical implications of this technology and to ensure that it is used in a responsible and ethical manner.。
基因克隆英文作文

基因克隆英文作文Cloning genes is a fascinating process that allows scientists to create identical copies of a particular gene. It involves isolating a specific gene from an organism's DNA and then making multiple copies of it using a technique called polymerase chain reaction (PCR).The potential applications of gene cloning are vast and diverse. For example, it can be used to produce large quantities of a particular protein for medical orindustrial purposes, or to create genetically modified organisms with desirable traits.However, gene cloning also raises ethical concerns, particularly when it comes to cloning humans. The idea of creating genetically identical copies of individuals raises questions about individuality, identity, and the potential for exploitation and abuse.Despite the ethical concerns, gene cloning continues tobe a valuable tool in scientific research. It has revolutionized fields such as biotechnology, medicine, and agriculture, and has the potential to continue making significant contributions to our understanding of genetics and the natural world.In conclusion, gene cloning is a powerful and controversial technology that has the potential to revolutionize the way we understand and manipulate the genetic code of living organisms. Its applications arewide-ranging and its implications far-reaching, making it a topic of ongoing debate and discussion in the scientific community and beyond.。
cloning and characterization of microRNAs from Rice
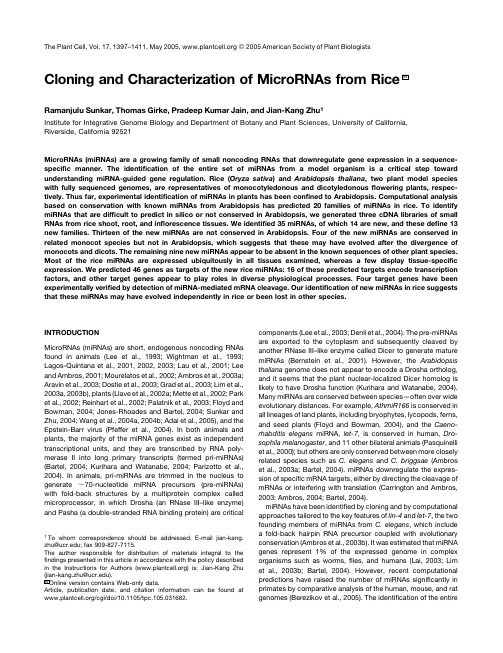
Cloning and Characterization of MicroRNAs from Rice WRamanjulu Sunkar,Thomas Girke,Pradeep Kumar Jain,and Jian-Kang Zhu1Institute for Integrative Genome Biology and Department of Botany and Plant Sciences,University of California,Riverside,California92521MicroRNAs(miRNAs)are a growing family of small noncoding RNAs that downregulate gene expression in a sequence-specific manner.The identification of the entire set of miRNAs from a model organism is a critical step toward understanding miRNA-guided gene regulation.Rice(Oryza sativa)and Arabidopsis thaliana,two plant model species with fully sequenced genomes,are representatives of monocotyledonous and dicotyledonousflowering plants,respec-tively.Thus far,experimental identification of miRNAs in plants has been confined to putational analysis based on conservation with known miRNAs from Arabidopsis has predicted20families of miRNAs in rice.To identify miRNAs that are difficult to predict in silico or not conserved in Arabidopsis,we generated three cDNA libraries of small RNAs from rice shoot,root,and inflorescence tissues.We identified35miRNAs,of which14are new,and these define13 new families.Thirteen of the new miRNAs are not conserved in Arabidopsis.Four of the new miRNAs are conserved in related monocot species but not in Arabidopsis,which suggests that these may have evolved after the divergence of monocots and dicots.The remaining nine new miRNAs appear to be absent in the known sequences of other plant species. Most of the rice miRNAs are expressed ubiquitously in all tissues examined,whereas a few display tissue-specific expression.We predicted46genes as targets of the new rice miRNAs:16of these predicted targets encode transcription factors,and other target genes appear to play roles in diverse physiological processes.Four target genes have been experimentally verified by detection of miRNA-mediated mRNA cleavage.Our identification of new miRNAs in rice suggests that these miRNAs may have evolved independently in rice or been lost in other species.INTRODUCTIONMicroRNAs(miRNAs)are short,endogenous noncoding RNAs found in animals(Lee et al.,1993;Wightman et al.,1993; Lagos-Quintana et al.,2001,2002,2003;Lau et al.,2001;Lee and Ambros,2001;Mourelatos et al.,2002;Ambros et al.,2003a; Aravin et al.,2003;Dostie et al.,2003;Grad et al.,2003;Lim et al., 2003a,2003b),plants(Llave et al.,2002a;Mette et al.,2002;Park et al.,2002;Reinhart et al.,2002;Palatnik et al.,2003;Floyd and Bowman,2004;Jones-Rhoades and Bartel,2004;Sunkar and Zhu,2004;Wang et al.,2004a,2004b;Adai et al.,2005),and the Epstein-Barr virus(Pfeffer et al.,2004).In both animals and plants,the majority of the miRNA genes exist as independent transcriptional units,and they are transcribed by RNA poly-merase II into long primary transcripts(termed pri-miRNAs) (Bartel,2004;Kurihara and Watanabe,2004;Parizotto et al., 2004).In animals,pri-miRNAs are trimmed in the nucleus to generate;70-nucleotide miRNA precursors(pre-miRNAs) with fold-back structures by a multiprotein complex called microprocessor,in which Drosha(an RNase III–like enzyme) and Pasha(a double-stranded RNA binding protein)are critical components(Lee et al.,2003;Denli et al.,2004).The pre-miRNAs are exported to the cytoplasm and subsequently cleaved by another RNase III–like enzyme called Dicer to generate mature miRNAs(Bernstein et al.,2001).However,the Arabidopsis thaliana genome does not appear to encode a Drosha ortholog, and it seems that the plant nuclear-localized Dicer homolog is likely to have Drosha function(Kurihara and Watanabe,2004). Many miRNAs are conserved between species—often over wide evolutionary distances.For example,AthmiR166is conserved in all lineages of land plants,including bryophytes,lycopods,ferns, and seed plants(Floyd and Bowman,2004),and the Caeno-rhabditis elegans miRNA,let-7,is conserved in human,Dro-sophila melanogaster,and11other bilateral animals(Pasquinelli et al.,2000);but others are only conserved between more closely related species such as C.elegans and C.briggsae(Ambros et al.,2003a;Bartel,2004).miRNAs downregulate the expres-sion of specific mRNA targets,either by directing the cleavage of mRNAs or interfering with translation(Carrington and Ambros, 2003;Ambros,2004;Bartel,2004).miRNAs have been identified by cloning and by computational approaches tailored to the key features of lin-4and let-7,the two founding members of miRNAs from C.elegans,which include a fold-back hairpin RNA precursor coupled with evolutionary conservation(Ambros et al.,2003b).It was estimated that miRNA genes represent1%of the expressed genome in complex organisms such as worms,flies,and humans(Lai,2003;Lim et al.,2003b;Bartel,2004).However,recent computational predictions have raised the number of miRNAs significantly in primates by comparative analysis of the human,mouse,and rat genomes(Berezikov et al.,2005).The identification of the entire1To whom correspondence should be addressed.E-mail jian-kang.zhu@;fax909-827-7115.The author responsible for distribution of materials integral to thefindings presented in this article in accordance with the policy describedin the Instructions for Authors()is:Jian-Kang Zhu(jian-kang.zhu@).W Online version contains Web-only data.Article,publication date,and citation information can be found at/cgi/doi/10.1105/tpc.105.031682.The Plant Cell,Vol.17,1397–1411,May2005,ª2005American Society of Plant Biologistsset of miRNAs and their target genes from model organisms is of fundamental importance to understand regulatory networks and gene silencing mechanisms.Rice(Oryza sativa)is the world’s most important crop,as measured by the portion of calories it provides to the human diet. It is an established model system for monocots that includes all cereals.Rice is the only monocot species with a fully sequenced genome.The availability of the complete genome sequence of rice allowed the in silico identification of20families of rice miRNAs based on conservation of sequences with Arabidopsis miRNAs(Park et al.,2002;Reinhart et al.,2002;Bonnet et al., 2004;Jones-Rhoades and Bartel,2004;Sunkar and Zhu,2004; Wang et al.,2004a;Adai et al.,2005).In addition tofinding conserved miRNAs,cloning approaches revealed Arabidopsis miRNAs that are not conserved in rice.At least four well-characterized Arabidopsis miRNAs,miR158,miR161,miR163, and miR173,do not have homologs in rice(Jones-Rhoades and Bartel,2004).Another miRNA(miR403)has been found to be conserved between Arabidopsis and Populus,whereas its counterpart could not be identified in rice(Sunkar and Zhu, 2004).Recently,evidence was shown that the nonconserved miR161and miR163from Arabidopsis may have evolved by inverted duplication of their target genes(Allen et al.,2004). Additionally,Berezikov et al.(2005)have predicted lineage-specific miRNAs in mammalian and nonmammalian animal species.Taken together,these observations support the notion that rice may express monocot-and/or rice-specific miRNAs. This study was undertaken to identify new miRNAs that are difficult to predict in silico and verify previously predicted miRNAs from rice.Sequencing of small RNA libraries and sub-sequent analysis led to the identification of14new miRNAs. These new miRNAs from rice form14families,13of which are new and not present in Arabidopsis.Furthermore,we confirmed the existence of15of the20conserved families of miRNAs that were predicted previously.Based on sequence complementarity to miRNAs,we were able to predict46rice genes as putative targets of the new miRNAs.These predicted targets include not only transcription factors but also other genes involved in diverse physiological processes.RESULTSIdentification of13New Families of Rice miRNAsBecause the available computational approaches can only identify miRNAs that are conserved between Arabidopsis and rice,a cloning approach was employed to identify rice miRNAs that may not be conserved or may have atypical features(Dugas and Bartel,2004).To this end,we generated three independent small RNA libraries from rice in the size range of18to26 nucleotides,from the shoots and roots of seedlings and in-florescence tissues of adult plants(O.sativa spp japonica cv Nipponbare).Small RNAs were isolated by size fractionation, ligated to59and39adapters,cloned,and sequenced.A total of ;10,000clones were sequenced(approximately one-third from each library),of which;5000small cDNA sequences were between18and26nucleotides in length.The remaining se-quences had either shorter fragments or self-ligated adapters. BLASTN searches revealed that97%of these sequences have at least one match in the rice nuclear genome sequence version 3annotated by The Institute for Genomic Research(TIGR) ().The remaining3%did not have a match and were not analyzed further.The lack of a match of these sequences may be due to unfinished regions in the rice genome sequence,sequencing errors,or other possibilities.Several clones were mapped to chloroplast or mitochondrial genomes and may represent either degradation or possibly regulatory products of organellar RNAs.The largest class of cloned RNAs represents fragments of abundant noncoding RNAs(rRNA, tRNA,small nuclear RNA,and small nucleolar RNA)as deter-mined by BLASTN searches against the Rfam database.A small fraction represents mRNA breakdown products from rice.The remaining sequences constitute miRNAs(Tables1and2)and endogenous small interfering RNAs(siRNAs)(data not shown). For95%of the endogenous siRNAs,we could not detect their expression on small RNA gel blots.miRNAs were distinguished from endogenous siRNAs on the basis of the ability of the miRNA surrounding sequences to adopt a hairpin structure(see Supplemental Figure1online). This analysis revealed that we had cloned35rice miRNAs.We also found one small RNA sequence that corresponds to OsmiR399g*.Sequence similarity searches against the central miRNA registry(/Software/Rfam/mirna/ search.shtml)showed that14of the miRNAs are new(Table1). The remaining21(belonging to15families)were identical with previously predicted miRNAs in rice(Table2).The newly iden-tified14miRNAs correspond to34loci.These new miRNAs belong to two predominant size classes:21and24nucleotides in length(Figure1).Nine of the14newly identified miRNAs begin with a59uridine,which is a characteristic feature of miRNAs (Table1).All14new miRNAs are perfectly conserved in Indica rice(O.sativa spp indica).One of the newly identified miRNAs is represented by two genomic loci,OsmiR396d and OsmiR396e,and is a member of previously predicted OsmiR396family in rice(Table1,Figure2). OsmiR396d was represented by10clones in our libraries and differed slightly in sequence from that of predicted OsmiR396 (Figure2).The predicted OsmiR396has three genomic loci (OsmiR396a,b,and c)and is represented by two members (Jones-Rhoades and Bartel,2004).OsmiR396d differs from these two members by the presence of an additional nucleotide G between ing a specific probe,we detected the expression of miR396d in rice and maize(Zea mays)but not in Arabidopsis(Figure7A).Although cross-hybridization often occurs between members in the same miRNA family,this is prevented by the presence of an additional nucleotide in the middle of miR396d.Consistent with the absence of a signal in the Arabidopsis RNA gel blots,the miR396d sequence is not pre-sent in the Arabidopsis genome.OsmiR396d sequence and the secondary structure of its precursor sequences are conserved in barley(Hordeum vulgare),another monocotolydonous plant (data not shown).Our sequence analysis indicated that we also identified a new miRNA that is conserved between monocots and dicots. We identified miR390in rice through cloning,whereas the1398The Plant CellArabidopsis counterpart was predicted through recent compu-tational approaches(Bonnet et al.,2004;Wang et al.,2004b). miR390is represented by one member with one locus in rice (Table1),whereas in Arabidopsis and Populus,it is represented by two members with three and six loci,respectively(see Supplemental Figure2online).Genomic Organization of the New Rice miRNAsGenomic locations of the new miRNA genes in rice are shown in Table1.The14newly identified miRNAs correspond to34loci.Hairpin structures can be predicted for all these34loci using miRNA surrounding sequences(see Supplemental Figure1 online).Ten of these are encoded by single copy miRNA genes, whereas the other four(miR439,miR396d,miR441,and miR445) have multiple loci in the genome(Table1).The exact origins of miRNAs corresponding to multiple genomic loci cannot be assigned unambiguously,and some of the loci could be pseu-dogenes.Our analysis of the genomic positions of the new miRNA genes shows that the majority localizes to intergenic regions(25out of34loci).However,seven correspond to introns of protein-coding genes in either the sense(6)or antisense(1)Table1.New Rice miRNAs Identified by CloningmiRNA Sequence(59/39)RNABlotSize(nt)No.ofLoci Chr.Fold-Back(59/39)Intergenic/Intron/Exon Osi Zm At PtmiR390AAGCUCAGGAGGGAUAGCGCC(2)þ211359IntergenicþþþþmiR396d UCCACAGGCUUUCUUGAACUG(10)þ212459IntergenicþþÿÿmiR396e259IntergenicmiR435UUAUCCGGUAUUGGAGUUGA(2)þ20133911669.m03227_Intron_7(s)þÿÿÿmiR436UGAGAGAGUGCACUUUCUCCC(2)þ211259J023035E19(s)þÿÿÿmiR437AAAGUUAGAGAAGUUUGACUU(2)þ21123911668.m02729_Intron_15(s)þþÿÿmiR438UUCCCACGCGUUAUAGUGAAA(1)þ211659IntergenicþÿÿÿmiR439a UGUCGAACCGCG GUUGUUCGA(1)þ2110139IntergenicþÿÿÿmiR439b1039IntergenicmiR439c139IntergenicmiR439d339IntergenicmiR439e739IntergenicmiR439f839IntergenicmiR439g839IntergenicmiR439h639IntergenicmiR439i939IntergenicmiR439j103911676.m02626_Intron_1(s)miR440AGUGUCUCCUGAUGAUCGGGACAA(2)þ241113911687.m01522_Intron_9(s)þÿÿÿmiR441a UACCAUCAAUAUAAAUGUGGGAAA(3)þ243139IntergenicþÿÿÿmiR441b339IntergenicmiR441c739IntergenicmiR442UGACGUGUAAAUUGCGAGACGAAU(2)þ24145911670.m05347_Intron_1(s)þÿÿÿmiR443AUCACAAUACAAUAAAUCUGGA(2)þ221359intergenicþÿÿÿmiR444UUGCUGCCUCAAGCUUGCUGC(3)þ211839J033125N22(s)þþÿÿmiR445a UAAAUUAGUGUAUAAACAUCCGAU(2)þ249539IntergenicþþÿÿmiR445b639IntergenicmiR445c1239IntergenicmiR445d339IntergenicmiR445e739IntergenicmiR445f339IntergenicmiR445g639IntergenicmiR445h33911669.m00786_Intron_2(as)miR445i63911680.m00766_Intron_1(s)miR446CAUCAAUAUGAAUAUGGGAAAUGG(2)þ241639IntergenicþÿÿÿFrequency of cloning is indicated in parentheses after the miRNA sequences.Chromosomal(Chr.)position is indicated.miRNA location in the predicted fold-back structure is specified(59or39arm).miRNAs that are conserved in other plants are indicated.Osi,O.sativa subspecies indica;Zm, Z.mays;At,A.thaliana;Pt,P.trichocarpa.nt,nucleotides.Rice miRNAs1399orientation(Table1).Our characterization of intronic origins of miRNAs was based on the latest annotation of the O.sativa spp japonica genome(version3.0).Two miRNAs(miR439and miR445)map to both intergenic and intronic locations.Two (miR435and miR440)are derived from introns only.Another two miRNAs(miR436and miR444)originate from the exons of protein-coding genes in the sense polarity(Table1). Biogenesis of OsmiR436and OsmiR444In general,most of the20-to25-nucleotide mature miRNAs are processed from a70-to300-nucleotide precursor,forming a hairpin structure that contains mature miRNA in either of its arms.Two miRNAs,miR436and miR444,were mapped to the exons of the protein-coding genes J023035E19(AK120922)and J033125N22(AK103332),respectively,in the sense polarity (Table1).The existence of these processed transcripts is sup-ported by expression data(Kikuchi et al.,2003).Both of the pre-cursor transcripts can form hairpin structures,and the miRNAs were detected on small RNA gel blots as discrete bands,sug-gesting that these are not nonspecific degradation products. The biogenesis of OsmiR444and OsmiR436is unusual be-cause the fold-back structure could not be predicted directly from the genomic sequence surrounding the miRNA.A hairpin structure can be predicted for a processed transcript(part of exons2and3sequences)but not with the genomic sequence, which suggests that the presence of an intron prevented the identification of a fold-back structure in the genomic locus of miR444(Figure3).The mature miR444resides in the3rd exon of the gene J033125N22.The open reading frame(ORF)of this gene is predicted to code for an unknown protein of50amino acids.It is possible though that the processed mRNA is just a pri-miRNA444transcript and does not code for a protein.miR444is conserved in monocots such as wheat(Triticum aestivum),barley,maize,sorghum(Sorghum bicolor),and sug-arcane(Saccharum officinarum)but not in Arabidopsis(Figure 4A).The precursor sequences from all these plants can form a hairpin structure(Figure4B).Unlike the situation in rice,the corresponding miRNA precursor fold-back structures can be predicted from the unspliced genomic sequences from the available sequences of other monocots.Similarly,OsmiR436also resides in the same polarity of a processed transcript(J023035E19),and only the processed transcript can form a hairpin structure.The mature miR436 resides in the3rd exon of the gene J023035E19.The predicted fold-back structure requires a very long part(720nucleotides; exons3to9)of the processed transcript(Figure5)because of the presence of stem-loop structures protruding from the39arm of the hairpin structure.Expression Patterns of Rice miRNAsThe tissue-and development-specific expression of miRNAs might provide clues about their physiological function.In a wideTable2.Experimental Verification of Previously Predicted miRNAs in RiceGene miRNA Sequence(59/39)Cloning Frequenciesin LibrariesOsmiR156a-j UGACAGAAGAGAGU-GAGCACShoot(12),root(5)OsmiR156k,l UGACAGAAGAGAGU-GAGCACA Shoot(3),root(1), inflorescence(1)OsmiR159a,b UUUGGAUUGAAGGG-AGCUCUShoot(3),inflorescence(2)OsmiR159c UUGGAUUGAAGGGA-GCUCUGCShoot(2)OsmiR160UGCCUGGCUCCCUG-UAUGCCAShoot(1)OsmiR164AUGGAGAAGCAGGG-CACGUGCAShoot(3),inflorescence(3)OsmiR166a-f UCGGACCAGGCUUC-AUUCCCCShoot(2),inflorescence(1)OsmiR167 a,b,c UGAAGCUGCCAGCA-UGAUCUAShoot(2)OsmiR167d-I UGAAGCUGCCAGCA-UGAUCUGRoot(1)OsmiR168a UCGCUUGGUGCAG-AUCGGGAC Shoot(14),root(3), inflorescence(5)OsmiR169b,c CAGCCAAGGAUGAC-UUGCCGGShoot(2)OsmiR169f,g UAGCCAAGGAUGAC-UUGCCUAShoot(2),root(1)OsmiR169 h-m UAGCCAAGGAUGAC-UUGCCUGShoot(1)OsmiR171a-f UGAUUGAGCCGUG-CCAAUAUC Shoot(1),root(1), inflorescence(2)OsmiR171g GAGGUGAGCCGUG-CCAAUAUCRoot(1)OsmiR172a AGAAUCUUGAUGAU-GCUGCAUShoot(2),inflorescence(2)OsmiR393UCCAAAGGGAUCGC-AUUGAUCInflorescence(1)OsmiR397b UUAUUGAGUGCAGC-GUUGAUGShoot(1),root(1)OsmiR398UGUGUUCUCAGGUC-GCCCCUGShoot(1)OsmiR399a UGCCAAAGGAGAAU-UGCCCUGShoot(1)OsmiR408CUGCACUGCCUCUU-CCCUGGCRoot(1)Figure1.Size Distribution of New miRNAs Cloned from Rice. 1400The Plant Cellrange of organisms,many miRNAs have been found to be differentially expressed at different developmental stages,cell types,and tissues (Lee and Ambros,2001;Lagos-Quintana et al.,2002;Aravin et al.,2003;Houbaviy et al.,2003).Several Arabidopsis miRNAs are expressed ubiquitously,whereas the expression of many others is regulated by development and shows preferential accumulation in certain tissues (Llave et al.,2002a;Park et al.,2002;Reinhart et al.,2002;Jones-Rhoades and Bartel,2004;Sunkar and Zhu,2004).To assist with the determination of the function of the new rice miRNAs,we examined their expression in different organs and developmental stages (Figures 6and 7).The expression patterns of miR441and miR442are similar:moderate expression in leaves,roots,and young seedlings and weaker expression in stems and inflorescences (Figures 6A and 6B).miR435and miR437also displayed similar expression patterns:moderate expression in leaves and young seedlings and weaker expression in other tissues tested (Figures 6C and6D).miR443and miR446seem to be strongly expressed in leaves and roots and moderately in stem and inflorescence tissues (Figures 6E and 6F).miR436is expressed in all tissues tested but the levels are higher in inflorescence and young seedlings (Figure 6G).miR438expression was moderate in leaves,root,inflorescence,and young seedlings (Figure 6H).miR440is uniformly and abundantly expressed in all rice tissues (Figure 6I),whereas miR439shows very weak expression in seedlings and is barely detectable in other tissues (Figure 6J).OsmiR396d showed strong and ubiquitous expression in all tissues,although the expression in roots was relatively lower (Figure 7A).miR445was strongly expressed in mature stems but barely detected in leaf and inflorescence tissues (Figure 7B).OsmiR444showed uniform expression in all rice tissues exam-ined (Figure 7C).All three miRNAs (miR396d,miR444,and miR445)were found to be expressed in maize,although the signal was weaker,possibly because of pooled RNA from different tissues of maize (Figures 7A to 7C).Some miRNAs displayed tissue-specific or developmental stage–specific expression patterns.Particularly interesting ex-pression was observed for miR445and miR390,which showed preferential expression in stems and roots,respectively (Figures 7B and 7D).To help determine whether a miRNA is conserved within monocots,its expression was analyzed in another monocot,maize.The analysis showed that three miRNAs (miR396d,miR444,and miR445)are conserved and expressed in maize (Figures 7A to 7C).The presence of miR396d and miR445miRNAs in other monocots is supported by their expressioninFigure 2.OsmiR396d Is a Unique Member of the Previously Predicted OsmiR396Family in Rice.Sequence alignment of miR396d and predicted members of OsmiR396family.Bold letter represents the additional nucleotide (nt)in the newmiRNA.Figure 3.Schematic Representation of the Biogenesis of OsmiR444.The hairpin structure requires parts of exon 2and exon 3of the host transcript J033125N22.Rice miRNAs 1401maize,and miR444by sequence and expression.In addition,miR437sequence and conserved precursor fold-back structures are present in maize,sugarcane,and sorghum (see Supplemen-tal Figure 3online).The absence of sequence and expression of these four miRNAs in Arabidopsis suggests that they may be specific to monocots.As an example of conserved miRNAs between dicots and monocots,we tested the expression of a newly identified and conserved miRNA,miR390,in addition to the previously reported and conserved OsmiR156and OsmiR171miRNAs in rice,maize,and Arabidopsis (Figures 7D to 7F).In addition to the expected size,the OsmiR171probe also hybridized to a slightly larger (;23bp)small RNA species in maize but not in rice or Arabidopsis,indicating that this larger species is specific to maize (Figure 7E).The remaining nine new rice miRNAs appear not to be conserved between plantspecies because they have no counterparts in other known plant sequences and their expression could not be detected in maize or Arabidopsis.In summary,the RNA gel blot analysis confirmed the expres-sion and sizes of 14newly identified miRNAs in rice (Figures 6and 7).The majority is expressed ubiquitously in all tissues.Predicted TargetsPrediction of plant miRNA targets has been facilitated by their extensive sequence complementarity (Rhoades et al.,2002;Bonnet et al.,2004;Jones-Rhoades and Bartel,2004;Sunkar and Zhu,2004;Wang et al.,2004b;Adai et al.,2005).Regulatory targets can be more confidently predicted for conserved miRNAs because complementary sites often are conservedacrossFigure 4.The miR444Family Is Conserved in Monocots.(A)Alignment of miR444sequence from rice with the predicted homologs in wheat,barley,maize,sorghum,and sugarcane.(B)Predicted fold-back structures of miR444precursors from rice,wheat,barley,maize,sorghum,and sugarcane.1402The Plant Cellspecies boundaries.To identify the potential targets of our newly identified miRNAs,we used the miRNA sequences to search the rice mRNA sequences for antisense hits with the PATSCAN program (Dsouza et al.,1997).Based on transcriptome analysis in Arabidopsis transgenic plants overexpressing miRNAs,Weigel and colleagues (Schwab et al.,2005)devised a set of rules for predicting miRNA targets.These criteria include allowing one mismatch in the region complementary to nucleotides 2to 12of the miRNA but not at the cleaving site (10and 11nucleotides),and three additional mismatches were permitted between 12-and 21-nucleotide positions,but no more than two continuous mis-matches within this region.Adopting these rules in predicting newly identified miRNA targets in rice,we allowed one mismatch between the positions 1to 9nucleotides from the 59end of miRNA and no mismatches between positions 10and 11,and another two mismatches were allowed between positions 12and 21/24.Gaps and mismatches are commonly seen in known animal and plant miRNA-mRNA basepairing interactions that are known to lead to cleavage or attenuation of translation (Aukerman and Sakai,2003;Palatnik et al.,2003;Chen,2004;Jones-Rhoades and Bartel,2004).By applying the above rules,our analysis led to the pre-diction of 46genes as putative targets for 11new miRNAs in rice (Table 3).Predicted targets and their complementarity with the new miRNAs are provided in Supplemental Table 1online.The number of predicted targets per miRNA varied greatly,from 1to 15.Four of the miRNAs (miR435,miR443,miR444,and miR445)each has only one predicted target.We were unable to predict targets for the remaining three miRNAs (miR438,miR440,and miR442)by applying these criteria.To evaluate the false positive rates of our target predictions,we performed the same searches with 100randomized sequences for each miRNA.The length and composition of the miRNAs were maintained in the randomized sets,and the searches were performed with the same mismatch settings (Rhoades et al.,2002;Jones-Rhoades and Bartel,2004).According to these results (Table 3),the hit frequency with the authentic miRNAs is in most cases more than five times higher and relative.In animals,all known miRNA target sites were found in 39untranslated regions (UTRs)of protein coding genes,whereas in plants they are only occasionally in the 39UTRs but are pre-dominantly in the coding regions (Rhoades et al.,2002;Bonnet et al.,2004;Jones-Rhoades and Bartel,2004;Sunkar and Zhu,2004;Wang et al.,2004b;Adai et al.,2005).In plants,they also have been predicted to reside in 59UTRs (Sunkar and Zhu,2004).Recently,miRNAs have also been predicted to target ORFs in humans (Lewis et al.,2005).Consistent with the earlier findings inArabidopsis,30of our predicted target genes in rice have target sites in their ORFs.Fifteen genes have their predicted target sites in 39UTR and only one in the 59UTR.Both miR444and its predicted target,a MADS box transcrip-tion factor gene,are conserved in other monocots,such as wheat,maize,barley,and sugarcane,but not in Arabidopsis (data not shown).OsmiR396d,a new member of the miR396family,is expected to target the growth-regulating factors (GRFs)(Jones-Rhoades and Bartel,2004).miR396d displays near perfect complementarity with 15of these GRF genes (Table 3;see Supplemental Table 1online).The complementary sites of miR396d are highly conserved in GRF genes of sorghum,maize,barley,and sugarcane.The predicted target of miR439,11667.m02576,a dirigent-like protein gene,was found to have three complementary sites within its ORF (Figure 8).These three sites are very closely spaced and separated by gaps of 11and 9nucleotides.One target site corresponding to the positions 567to 588is perfectly complementary to the miRNA.The other two target sites corre-spond to the positions 599to 619and 628to 648,with two and four mismatches,respectively (Figure 8).The two target sites corresponding to positions 567to 588and 628to 648are in frame,and there is partial amino acid sequence conservation between these two target sites (Figure 8).The presence of three target sites within one ORF has not been seen before and thus is unique among miRNA targets predicted thus far.It appears that our predicted targets play roles not only in development but also in diverse physiological processes.Six-teen of the predicted targets of two miRNAs (miR396d and miR444)are transcription factors (GRFs and MADS box tran-scription factors),whereas the remaining 30predicted targets of nine miRNAs appear to play roles in a broad range of physiolog-ical processes and include protein kinases,F-box proteins,dirigent-like protein,Glu receptor-like proteins,RNA binding protein,retrotransposons,and 17other proteins with unknown function (Table 3).Identification of miRNA-Guided Cleavage of Target mRNAs in RicemiRNAs negatively regulate target genes through miRNA-directed cleavage within the region of complementarity or inter-fering with translation (Carrington and Ambros,2003;Ambros,2004;Bartel,2004;Dugas and Bartel,2004;Mallory and Vaucheret,2004).Most of the Arabidopsis miRNAs have been shown to guide the cleavage of target mRNAs(LlaveFigure 5.Predicted Fold-Back Structure of OsmiR436Precursor.The fold-back structure was predicted with use of a 720-bp processed transcript.Protruding stem-loops in the 39arm of the hairpin are indicated by the slashes (\).Rice miRNAs 1403。
分子生物学英文文献6

Chapter19Detection and Quantitative Analysis of Small RNAs by PCR Seungil Ro and Wei YanAbstractIncreasing lines of evidence indicate that small non-coding RNAs including miRNAs,piRNAs,rasiRNAs, 21U endo-siRNAs,and snoRNAs are involved in many critical biological processes.Functional studies of these small RNAs require a simple,sensitive,and reliable method for detecting and quantifying levels of small RNAs.Here,we describe such a method that has been widely used for the validation of cloned small RNAs and also for quantitative analyses of small RNAs in both tissues and cells.Key words:Small RNAs,miRNAs,piRNAs,expression,PCR.1.IntroductionThe past several years have witnessed the surprising discovery ofnumerous non-coding small RNAs species encoded by genomesof virtually all species(1–6),which include microRNAs(miR-NAs)(7–10),piwi-interacting RNAs(piRNAs)(11–14),repeat-associated siRNAs(rasiRNAs)(15–18),21U endo-siRNAs(19),and small nucleolar RNAs(snoRNAs)(20).These small RNAsare involved in all aspects of cellular functions through direct orindirect interactions with genomic DNAs,RNAs,and proteins.Functional studies on these small RNAs are just beginning,andsome preliminaryfindings have suggested that they are involvedin regulating genome stability,epigenetic marking,transcription,translation,and protein functions(5,21–23).An easy and sensi-tive method to detect and quantify levels of these small RNAs inorgans or cells during developmental courses,or under different M.Sioud(ed.),RNA Therapeutics,Methods in Molecular Biology629,DOI10.1007/978-1-60761-657-3_19,©Springer Science+Business Media,LLC2010295296Ro and Yanphysiological and pathophysiological conditions,is essential forfunctional studies.Quantitative analyses of small RNAs appear tobe challenging because of their small sizes[∼20nucleotides(nt)for miRNAs,∼30nt for piRNAs,and60–200nt for snoRNAs].Northern blot analysis has been the standard method for detec-tion and quantitative analyses of RNAs.But it requires a relativelylarge amount of starting material(10–20μg of total RNA or>5μg of small RNA fraction).It is also a labor-intensive pro-cedure involving the use of polyacrylamide gel electrophoresis,electrotransfer,radioisotope-labeled probes,and autoradiogra-phy.We have developed a simple and reliable PCR-based methodfor detection and quantification of all types of small non-codingRNAs.In this method,small RNA fractions are isolated and polyAtails are added to the3 ends by polyadenylation(Fig.19.1).Small RNA cDNAs(srcDNAs)are then generated by reverseFig.19.1.Overview of small RNA complementary DNA(srcDNA)library construction forPCR or qPCR analysis.Small RNAs are polyadenylated using a polyA polymerase.ThepolyA-tailed RNAs are reverse-transcribed using a primer miRTQ containing oligo dTsflanked by an adaptor sequence.RNAs are removed by RNase H from the srcDNA.ThesrcDNA is ready for PCR or qPCR to be carried out using a small RNA-specific primer(srSP)and a universal reverse primer,RTQ-UNIr.Quantitative Analysis of Small RNAs297transcription using a primer consisting of adaptor sequences atthe5 end and polyT at the3 end(miRTQ).Using the srcD-NAs,non-quantitative or quantitative PCR can then be per-formed using a small RNA-specific primer and the RTQ-UNIrprimer.This method has been utilized by investigators in numer-ous studies(18,24–38).Two recent technologies,454sequenc-ing and microarray(39,40)for high-throughput analyses of miR-NAs and other small RNAs,also need an independent method forvalidation.454sequencing,the next-generation sequencing tech-nology,allows virtually exhaustive sequencing of all small RNAspecies within a small RNA library.However,each of the clonednovel small RNAs needs to be validated by examining its expres-sion in organs or in cells.Microarray assays of miRNAs have beenavailable but only known or bioinformatically predicted miR-NAs are covered.Similar to mRNA microarray analyses,the up-or down-regulation of miRNA levels under different conditionsneeds to be further validated using conventional Northern blotanalyses or PCR-based methods like the one that we are describ-ing here.2.Materials2.1.Isolation of Small RNAs, Polyadenylation,and Purification 1.mirVana miRNA Isolation Kit(Ambion).2.Phosphate-buffered saline(PBS)buffer.3.Poly(A)polymerase.4.mirVana Probe and Marker Kit(Ambion).2.2.Reverse Transcription,PCR, and Quantitative PCR 1.Superscript III First-Strand Synthesis System for RT-PCR(Invitrogen).2.miRTQ primers(Table19.1).3.AmpliTaq Gold PCR Master Mix for PCR.4.SYBR Green PCR Master Mix for qPCR.5.A miRNA-specific primer(e.g.,let-7a)and RTQ-UNIr(Table19.1).6.Agarose and100bp DNA ladder.3.Methods3.1.Isolation of Small RNAs 1.Harvest tissue(≤250mg)or cells in a1.7-mL tube with500μL of cold PBS.T a b l e 19.1O l i g o n u c l e o t i d e s u s e dN a m eS e q u e n c e (5 –3 )N o t eU s a g em i R T QC G A A T T C T A G A G C T C G A G G C A G G C G A C A T G G C T G G C T A G T T A A G C T T G G T A C C G A G C T A G T C C T T T T T T T T T T T T T T T T T T T T T T T T T V N ∗R N a s e f r e e ,H P L CR e v e r s e t r a n s c r i p t i o nR T Q -U N I r C G A A T T C T A G A G C T C G A G G C A G GR e g u l a r d e s a l t i n gP C R /q P C Rl e t -7a T G A G G T A G T A G G T T G T A T A G R e g u l a r d e s a l t i n gP C R /q P C R∗V =A ,C ,o r G ;N =A ,C ,G ,o r TQuantitative Analysis of Small RNAs299 2.Centrifuge at∼5,000rpm for2min at room temperature(RT).3.Remove PBS as much as possible.For cells,remove PBScarefully without breaking the pellet,leave∼100μL of PBS,and resuspend cells by tapping gently.4.Add300–600μL of lysis/binding buffer(10volumes pertissue mass)on ice.When you start with frozen tissue or cells,immediately add lysis/binding buffer(10volumes per tissue mass)on ice.5.Cut tissue into small pieces using scissors and grind it usinga homogenizer.For cells,skip this step.6.Vortex for40s to mix.7.Add one-tenth volume of miRNA homogenate additive onice and mix well by vortexing.8.Leave the mixture on ice for10min.For tissue,mix it every2min.9.Add an equal volume(330–660μL)of acid-phenol:chloroform.Be sure to withdraw from the bottom phase(the upper phase is an aqueous buffer).10.Mix thoroughly by inverting the tubes several times.11.Centrifuge at10,000rpm for5min at RT.12.Recover the aqueous phase carefully without disrupting thelower phase and transfer it to a fresh tube.13.Measure the volume using a scale(1g=∼1mL)andnote it.14.Add one-third volume of100%ethanol at RT to the recov-ered aqueous phase.15.Mix thoroughly by inverting the tubes several times.16.Transfer up to700μL of the mixture into afilter cartridgewithin a collection bel thefilter as total RNA.When you have>700μL of the mixture,apply it in suc-cessive application to the samefilter.17.Centrifuge at10,000rpm for15s at RT.18.Collect thefiltrate(theflow-through).Save the cartridgefor total RNA isolation(go to Step24).19.Add two-third volume of100%ethanol at RT to theflow-through.20.Mix thoroughly by inverting the tubes several times.21.Transfer up to700μL of the mixture into a newfilterbel thefilter as small RNA.When you have >700μL of thefiltrate mixture,apply it in successive appli-cation to the samefilter.300Ro and Yan22.Centrifuge at10,000rpm for15s at RT.23.Discard theflow-through and repeat until all of thefiltratemixture is passed through thefilter.Reuse the collectiontube for the following washing steps.24.Apply700μL of miRNA wash solution1(working solu-tion mixed with ethanol)to thefilter.25.Centrifuge at10,000rpm for15s at RT.26.Discard theflow-through.27.Apply500μL of miRNA wash solution2/3(working solu-tion mixed with ethanol)to thefilter.28.Centrifuge at10,000rpm for15s at RT.29.Discard theflow-through and repeat Step27.30.Centrifuge at12,000rpm for1min at RT.31.Transfer thefilter cartridge to a new collection tube.32.Apply100μL of pre-heated(95◦C)elution solution orRNase-free water to the center of thefilter and close thecap.Aliquot a desired amount of elution solution intoa1.7-mL tube and heat it on a heat block at95◦C for∼15min.Open the cap carefully because it might splashdue to pressure buildup.33.Leave thefilter tube alone for1min at RT.34.Centrifuge at12,000rpm for1min at RT.35.Measure total RNA and small RNA concentrations usingNanoDrop or another spectrophotometer.36.Store it at–80◦C until used.3.2.Polyadenylation1.Set up a reaction mixture with a total volume of50μL in a0.5-mL tube containing0.1–2μg of small RNAs,10μL of5×E-PAP buffer,5μL of25mM MnCl2,5μL of10mMATP,1μL(2U)of Escherichia coli poly(A)polymerase I,and RNase-free water(up to50μL).When you have a lowconcentration of small RNAs,increase the total volume;5×E-PAP buffer,25mM MnCl2,and10mM ATP should beincreased accordingly.2.Mix well and spin the tube briefly.3.Incubate for1h at37◦C.3.3.Purification 1.Add an equal volume(50μL)of acid-phenol:chloroformto the polyadenylation reaction mixture.When you have>50μL of the mixture,increase acid-phenol:chloroformaccordingly.2.Mix thoroughly by tapping the tube.Quantitative Analysis of Small RNAs3013.Centrifuge at10,000rpm for5min at RT.4.Recover the aqueous phase carefully without disrupting thelower phase and transfer it to a fresh tube.5.Add12volumes(600μL)of binding/washing buffer tothe aqueous phase.When you have>50μL of the aqueous phase,increase binding/washing buffer accordingly.6.Transfer up to460μL of the mixture into a purificationcartridge within a collection tube.7.Centrifuge at10,000rpm for15s at RT.8.Discard thefiltrate(theflow-through)and repeat until allof the mixture is passed through the cartridge.Reuse the collection tube.9.Apply300μL of binding/washing buffer to the cartridge.10.Centrifuge at12,000rpm for1min at RT.11.Transfer the cartridge to a new collection tube.12.Apply25μL of pre-heated(95◦C)elution solution to thecenter of thefilter and close the cap.Aliquot a desired amount of elution solution into a1.7-mL tube and heat it on a heat block at95◦C for∼15min.Open the cap care-fully because it might be splash due to pressure buildup.13.Let thefilter tube stand for1min at RT.14.Centrifuge at12,000rpm for1min at RT.15.Repeat Steps12–14with a second aliquot of25μL ofpre-heated(95◦C)elution solution.16.Measure polyadenylated(tailed)RNA concentration usingNanoDrop or another spectrophotometer.17.Store it at–80◦C until used.After polyadenylation,RNAconcentration should increase up to5–10times of the start-ing concentration.3.4.Reverse Transcription 1.Mix2μg of tailed RNAs,1μL(1μg)of miRTQ,andRNase-free water(up to21μL)in a PCR tube.2.Incubate for10min at65◦C and for5min at4◦C.3.Add1μL of10mM dNTP mix,1μL of RNaseOUT,4μLof10×RT buffer,4μL of0.1M DTT,8μL of25mM MgCl2,and1μL of SuperScript III reverse transcriptase to the mixture.When you have a low concentration of lig-ated RNAs,increase the total volume;10×RT buffer,0.1M DTT,and25mM MgCl2should be increased accordingly.4.Mix well and spin the tube briefly.5.Incubate for60min at50◦C and for5min at85◦C toinactivate the reaction.302Ro and Yan6.Add1μL of RNase H to the mixture.7.Incubate for20min at37◦C.8.Add60μL of nuclease-free water.3.5.PCR and qPCR 1.Set up a reaction mixture with a total volume of25μL ina PCR tube containing1μL of small RNA cDNAs(srcD-NAs),1μL(5pmol of a miRNA-specific primer(srSP),1μL(5pmol)of RTQ-UNIr,12.5μL of AmpliTaq GoldPCR Master Mix,and9.5μL of nuclease-free water.ForqPCR,use SYBR Green PCR Master Mix instead of Ampli-Taq Gold PCR Master Mix.2.Mix well and spin the tube briefly.3.Start PCR or qPCR with the conditions:95◦C for10minand then40cycles at95◦C for15s,at48◦C for30s and at60◦C for1min.4.Adjust annealing Tm according to the Tm of your primer5.Run2μL of the PCR or qPCR products along with a100bpDNA ladder on a2%agarose gel.∼PCR products should be∼120–200bp depending on the small RNA species(e.g.,∼120–130bp for miRNAs and piRNAs).4.Notes1.This PCR method can be used for quantitative PCR(qPCR)or semi-quantitative PCR(semi-qPCR)on small RNAs suchas miRNAs,piRNAs,snoRNAs,small interfering RNAs(siRNAs),transfer RNAs(tRNAs),and ribosomal RNAs(rRNAs)(18,24–38).2.Design miRNA-specific primers to contain only the“coresequence”since our cloning method uses two degeneratenucleotides(VN)at the3 end to make small RNA cDNAs(srcDNAs)(see let-7a,Table19.1).3.For qPCR analysis,two miRNAs and a piRNA were quan-titated using the SYBR Green PCR Master Mix(41).Cyclethreshold(Ct)is the cycle number at which thefluorescencesignal reaches the threshold level above the background.ACt value for each miRNA tested was automatically calculatedby setting the threshold level to be0.1–0.3with auto base-line.All Ct values depend on the abundance of target miR-NAs.For example,average Ct values for let-7isoforms rangefrom17to20when25ng of each srcDNA sample from themultiple tissues was used(see(41).Quantitative Analysis of Small RNAs3034.This method amplifies over a broad dynamic range up to10orders of magnitude and has excellent sensitivity capable ofdetecting as little as0.001ng of the srcDNA in qPCR assays.5.For qPCR,each small RNA-specific primer should be testedalong with a known control primer(e.g.,let-7a)for PCRefficiency.Good efficiencies range from90%to110%calcu-lated from slopes between–3.1and–3.6.6.On an agarose gel,mature miRNAs and precursor miRNAs(pre-miRNAs)can be differentiated by their size.PCR prod-ucts containing miRNAs will be∼120bp long in size whileproducts containing pre-miRNAs will be∼170bp long.However,our PCR method preferentially amplifies maturemiRNAs(see Results and Discussion in(41)).We testedour PCR method to quantify over100miRNAs,but neverdetected pre-miRNAs(18,29–31,38). AcknowledgmentsThe authors would like to thank Jonathan Cho for reading andediting the text.This work was supported by grants from theNational Institute of Health(HD048855and HD050281)toW.Y.References1.Ambros,V.(2004)The functions of animalmicroRNAs.Nature,431,350–355.2.Bartel,D.P.(2004)MicroRNAs:genomics,biogenesis,mechanism,and function.Cell, 116,281–297.3.Chang,T.C.and Mendell,J.T.(2007)Theroles of microRNAs in vertebrate physiol-ogy and human disease.Annu Rev Genomics Hum Genet.4.Kim,V.N.(2005)MicroRNA biogenesis:coordinated cropping and dicing.Nat Rev Mol Cell Biol,6,376–385.5.Kim,V.N.(2006)Small RNAs just gotbigger:Piwi-interacting RNAs(piRNAs) in mammalian testes.Genes Dev,20, 1993–1997.6.Kotaja,N.,Bhattacharyya,S.N.,Jaskiewicz,L.,Kimmins,S.,Parvinen,M.,Filipowicz, W.,and Sassone-Corsi,P.(2006)The chro-matoid body of male germ cells:similarity with processing bodies and presence of Dicer and microRNA pathway components.Proc Natl Acad Sci U S A,103,2647–2652.7.Aravin,A.A.,Lagos-Quintana,M.,Yalcin,A.,Zavolan,M.,Marks,D.,Snyder,B.,Gaaster-land,T.,Meyer,J.,and Tuschl,T.(2003) The small RNA profile during Drosophilamelanogaster development.Dev Cell,5, 337–350.8.Lee,R.C.and Ambros,V.(2001)An exten-sive class of small RNAs in Caenorhabditis ele-gans.Science,294,862–864.u,N.C.,Lim,L.P.,Weinstein, E.G.,and Bartel,D.P.(2001)An abundant class of tiny RNAs with probable regulatory roles in Caenorhabditis elegans.Science,294, 858–862.gos-Quintana,M.,Rauhut,R.,Lendeckel,W.,and Tuschl,T.(2001)Identification of novel genes coding for small expressed RNAs.Science,294,853–858.u,N.C.,Seto,A.G.,Kim,J.,Kuramochi-Miyagawa,S.,Nakano,T.,Bartel,D.P.,and Kingston,R.E.(2006)Characterization of the piRNA complex from rat testes.Science, 313,363–367.12.Grivna,S.T.,Beyret,E.,Wang,Z.,and Lin,H.(2006)A novel class of small RNAs inmouse spermatogenic cells.Genes Dev,20, 1709–1714.13.Girard, A.,Sachidanandam,R.,Hannon,G.J.,and Carmell,M.A.(2006)A germline-specific class of small RNAs binds mammalian Piwi proteins.Nature,442,199–202.304Ro and Yan14.Aravin,A.,Gaidatzis,D.,Pfeffer,S.,Lagos-Quintana,M.,Landgraf,P.,Iovino,N., Morris,P.,Brownstein,M.J.,Kuramochi-Miyagawa,S.,Nakano,T.,Chien,M.,Russo, J.J.,Ju,J.,Sheridan,R.,Sander,C.,Zavolan, M.,and Tuschl,T.(2006)A novel class of small RNAs bind to MILI protein in mouse testes.Nature,442,203–207.15.Watanabe,T.,Takeda, A.,Tsukiyama,T.,Mise,K.,Okuno,T.,Sasaki,H.,Minami, N.,and Imai,H.(2006)Identification and characterization of two novel classes of small RNAs in the mouse germline: retrotransposon-derived siRNAs in oocytes and germline small RNAs in testes.Genes Dev,20,1732–1743.16.Vagin,V.V.,Sigova,A.,Li,C.,Seitz,H.,Gvozdev,V.,and Zamore,P.D.(2006)A distinct small RNA pathway silences selfish genetic elements in the germline.Science, 313,320–324.17.Saito,K.,Nishida,K.M.,Mori,T.,Kawa-mura,Y.,Miyoshi,K.,Nagami,T.,Siomi,H.,and Siomi,M.C.(2006)Specific asso-ciation of Piwi with rasiRNAs derived from retrotransposon and heterochromatic regions in the Drosophila genome.Genes Dev,20, 2214–2222.18.Ro,S.,Song,R.,Park, C.,Zheng,H.,Sanders,K.M.,and Yan,W.(2007)Cloning and expression profiling of small RNAs expressed in the mouse ovary.RNA,13, 2366–2380.19.Ruby,J.G.,Jan,C.,Player,C.,Axtell,M.J.,Lee,W.,Nusbaum,C.,Ge,H.,and Bartel,D.P.(2006)Large-scale sequencing reveals21U-RNAs and additional microRNAs and endogenous siRNAs in C.elegans.Cell,127, 1193–1207.20.Terns,M.P.and Terns,R.M.(2002)Small nucleolar RNAs:versatile trans-acting molecules of ancient evolutionary origin.Gene Expr,10,17–39.21.Ouellet,D.L.,Perron,M.P.,Gobeil,L.A.,Plante,P.,and Provost,P.(2006)MicroR-NAs in gene regulation:when the smallest governs it all.J Biomed Biotechnol,2006, 69616.22.Maatouk,D.and Harfe,B.(2006)MicroR-NAs in development.ScientificWorldJournal, 6,1828–1840.23.Kim,V.N.and Nam,J.W.(2006)Genomics of microRNA.Trends Genet,22, 165–173.24.Bohnsack,M.T.,Kos,M.,and Tollervey,D.(2008)Quantitative analysis of snoRNAassociation with pre-ribosomes and release of snR30by Rok1helicase.EMBO Rep,9, 1230–1236.25.Hertel,J.,de Jong, D.,Marz,M.,Rose,D.,Tafer,H.,Tanzer, A.,Schierwater,B.,and Stadler,P.F.(2009)Non-codingRNA annotation of the genome of Tri-choplax adhaerens.Nucleic Acids Res,37, 1602–1615.26.Kim,M.,Patel,B.,Schroeder,K.E.,Raza,A.,and Dejong,J.(2008)Organization andtranscriptional output of a novel mRNA-like piRNA gene(mpiR)located on mouse chro-mosome10.RNA,14,1005–1011.27.Mishima,T.,Takizawa,T.,Luo,S.S.,Ishibashi,O.,Kawahigashi,Y.,Mizuguchi, Y.,Ishikawa,T.,Mori,M.,Kanda,T., and Goto,T.(2008)MicroRNA(miRNA) cloning analysis reveals sex differences in miRNA expression profiles between adult mouse testis and ovary.Reproduction,136, 811–822.28.Papaioannou,M.D.,Pitetti,J.L.,Ro,S.,Park, C.,Aubry, F.,Schaad,O.,Vejnar,C.E.,Kuhne, F.,Descombes,P.,Zdob-nov, E.M.,McManus,M.T.,Guillou, F., Harfe,B.D.,Yan,W.,Jegou,B.,and Nef, S.(2009)Sertoli cell Dicer is essential for spermatogenesis in mice.Dev Biol,326, 250–259.29.Ro,S.,Park,C.,Sanders,K.M.,McCarrey,J.R.,and Yan,W.(2007)Cloning and expres-sion profiling of testis-expressed microRNAs.Dev Biol,311,592–602.30.Ro,S.,Park,C.,Song,R.,Nguyen,D.,Jin,J.,Sanders,K.M.,McCarrey,J.R.,and Yan, W.(2007)Cloning and expression profiling of testis-expressed piRNA-like RNAs.RNA, 13,1693–1702.31.Ro,S.,Park,C.,Young,D.,Sanders,K.M.,and Yan,W.(2007)Tissue-dependent paired expression of miRNAs.Nucleic Acids Res, 35,5944–5953.32.Siebolts,U.,Varnholt,H.,Drebber,U.,Dienes,H.P.,Wickenhauser,C.,and Oden-thal,M.(2009)Tissues from routine pathol-ogy archives are suitable for microRNA anal-yses by quantitative PCR.J Clin Pathol,62, 84–88.33.Smits,G.,Mungall,A.J.,Griffiths-Jones,S.,Smith,P.,Beury,D.,Matthews,L.,Rogers, J.,Pask, A.J.,Shaw,G.,VandeBerg,J.L., McCarrey,J.R.,Renfree,M.B.,Reik,W.,and Dunham,I.(2008)Conservation of the H19 noncoding RNA and H19-IGF2imprint-ing mechanism in therians.Nat Genet,40, 971–976.34.Song,R.,Ro,S.,Michaels,J.D.,Park,C.,McCarrey,J.R.,and Yan,W.(2009)Many X-linked microRNAs escape meiotic sex chromosome inactivation.Nat Genet,41, 488–493.Quantitative Analysis of Small RNAs30535.Wang,W.X.,Wilfred,B.R.,Baldwin,D.A.,Isett,R.B.,Ren,N.,Stromberg, A.,and Nelson,P.T.(2008)Focus on RNA iso-lation:obtaining RNA for microRNA (miRNA)expression profiling analyses of neural tissue.Biochim Biophys Acta,1779, 749–757.36.Wu,F.,Zikusoka,M.,Trindade,A.,Das-sopoulos,T.,Harris,M.L.,Bayless,T.M., Brant,S.R.,Chakravarti,S.,and Kwon, J.H.(2008)MicroRNAs are differen-tially expressed in ulcerative colitis and alter expression of macrophage inflam-matory peptide-2alpha.Gastroenterology, 135(1624–1635),e24.37.Wu,H.,Neilson,J.R.,Kumar,P.,Manocha,M.,Shankar,P.,Sharp,P.A.,and Manjunath, N.(2007)miRNA profiling of naive,effec-tor and memory CD8T cells.PLoS ONE,2, e1020.38.Yan,W.,Morozumi,K.,Zhang,J.,Ro,S.,Park, C.,and Yanagimachi,R.(2008) Birth of mice after intracytoplasmic injec-tion of single purified sperm nuclei and detection of messenger RNAs and microR-NAs in the sperm nuclei.Biol Reprod,78, 896–902.39.Guryev,V.and Cuppen,E.(2009)Next-generation sequencing approaches in genetic rodent model systems to study func-tional effects of human genetic variation.FEBS Lett.40.Li,W.and Ruan,K.(2009)MicroRNAdetection by microarray.Anal Bioanal Chem.41.Ro,S.,Park,C.,Jin,JL.,Sanders,KM.,andYan,W.(2006)A PCR-based method for detection and quantification of small RNAs.Biochem and Biophys Res Commun,351, 756–763.。
微生物学名词解释

绪论微生物分类学microbial tasonomy 研究微生物分类理论和技术方法的学科称为微生物分类学。
分类classification 分类是根据一定的原则(表型特征相似性或系统发育相关性)对微生物进行分群归类,根据相似性或相关性水平排列成系统,并对各个分类群的特征进行描述,以便查考和对未被分类的微生物进行鉴定。
命名nomenclature 命名是根据命名法规,给每一个分类群一个专有的名称。
鉴定identification 指借助于现有的微生物分类系统,通过特征测定,确定未知的、新发现的或未明确分类地位微生物所应归属分类群的过程。
分类单元taxon, 复数taxa 是指具体的分类群,如原核生物界(Procaryotae)、肠杆菌科(Enterobacteriaceae)、枯草芽孢杆菌(Bacillus subtilis)等都分别代表一个分类单元。
种species 种是生物分类中基本的分类单元和分类等级。
微生物的种可以看作是:具有高度特征相似性的菌株群,这个菌株群与其他类群的菌株有很明显的区别。
属g enus 是介于种(或亚种)与科之间的分类等级,也是生物分类中的基本分类单元。
通常是把具有某些共同特征或密切相关的种归为一个高一级的分类单元,称之属。
.居群population 是指一定空间中同种个体的总和。
每一个物种早自然界中的存在,都有一定的空间结构,在其分散的、不连续的居住场所或分布区域内,形成不同的群体单元,这些群体单元就称居群。
亚种subspecies, subsp., ssp. 当某一工人种内的不同菌株存在少数明显而稳定的变异特征或遗传性状而又不足以区分成新种时,可以将这些菌株细分成两个或更多的小的分类单元称为亚种。
亚种是正式分类单元中地位最低的分类等级。
变种variety 变种是亚种的同义词。
在《国际细菌命名法规》(1976年修订本)发表以前,变种是种的亚等级,因“变种”一词易引起词义上的混淆,1976年后,细菌种的亚等级一律采用亚种,而不再使用变种。
A Versatile Zero Background T-Vector System for Gene
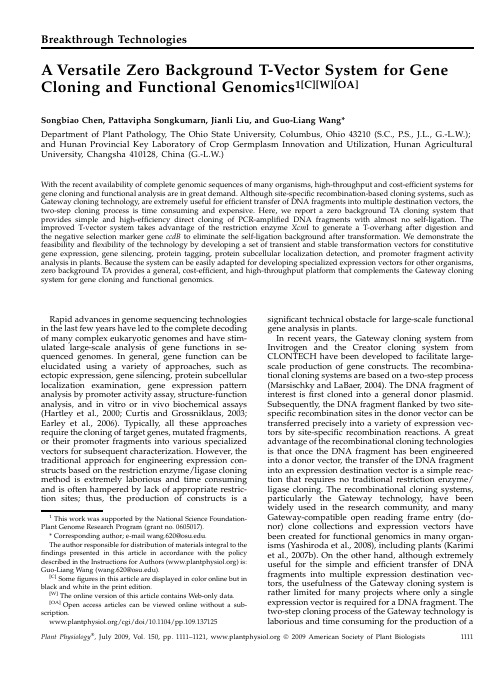
Breakthrough TechnologiesA Versatile Zero Background T-Vector System for Gene Cloning and Functional Genomics1[C][W][OA]Songbiao Chen,Pattavipha Songkumarn,Jianli Liu,and Guo-Liang Wang*Department of Plant Pathology,The Ohio State University,Columbus,Ohio43210(S.C.,P.S.,J.L.,G.-L.W.); and Hunan Provincial Key Laboratory of Crop Germplasm Innovation and Utilization,Hunan Agricultural University,Changsha410128,China(G.-L.W.)With the recent availability of complete genomic sequences of many organisms,high-throughput and cost-efficient systems for gene cloning and functional analysis are in great demand.Although site-specific recombination-based cloning systems,such as Gateway cloning technology,are extremely useful for efficient transfer of DNA fragments into multiple destination vectors,the two-step cloning process is time consuming and expensive.Here,we report a zero background TA cloning system that provides simple and high-efficiency direct cloning of PCR-amplified DNA fragments with almost no self-ligation.The improved T-vector system takes advantage of the restriction enzyme Xcm I to generate a T-overhang after digestion and the negative selection marker gene ccdB to eliminate the self-ligation background after transformation.We demonstrate the feasibility andflexibility of the technology by developing a set of transient and stable transformation vectors for constitutive gene expression,gene silencing,protein tagging,protein subcellular localization detection,and promoter fragment activity analysis in plants.Because the system can be easily adapted for developing specialized expression vectors for other organisms, zero background TA provides a general,cost-efficient,and high-throughput platform that complements the Gateway cloning system for gene cloning and functional genomics.Rapid advances in genome sequencing technologies in the last few years have led to the complete decoding of many complex eukaryotic genomes and have stim-ulated large-scale analysis of gene functions in se-quenced genomes.In general,gene function can be elucidated using a variety of approaches,such as ectopic expression,gene silencing,protein subcellular localization examination,gene expression pattern analysis by promoter activity assay,structure-function analysis,and in vitro or in vivo biochemical assays (Hartley et al.,2000;Curtis and Grossniklaus,2003; Earley et al.,2006).Typically,all these approaches require the cloning of target genes,mutated fragments, or their promoter fragments into various specialized vectors for subsequent characterization.However,the traditional approach for engineering expression con-structs based on the restriction enzyme/ligase cloning method is extremely laborious and time consuming and is often hampered by lack of appropriate restric-tion sites;thus,the production of constructs is a significant technical obstacle for large-scale functional gene analysis in plants.In recent years,the Gateway cloning system from Invitrogen and the Creator cloning system from CLONTECH have been developed to facilitate large-scale production of gene constructs.The recombina-tional cloning systems are based on a two-step process (Marsischky and LaBaer,2004).The DNA fragment of interest isfirst cloned into a general donor plasmid. Subsequently,the DNA fragmentflanked by two site-specific recombination sites in the donor vector can be transferred precisely into a variety of expression vec-tors by site-specific recombination reactions.A great advantage of the recombinational cloning technologies is that once the DNA fragment has been engineered into a donor vector,the transfer of the DNA fragment into an expression destination vector is a simple reac-tion that requires no traditional restriction enzyme/ ligase cloning.The recombinational cloning systems, particularly the Gateway technology,have been widely used in the research community,and many Gateway-compatible open reading frame entry(do-nor)clone collections and expression vectors have been created for functional genomics in many organ-isms(Yashiroda et al.,2008),including plants(Karimi et al.,2007b).On the other hand,although extremely useful for the simple and efficient transfer of DNA fragments into multiple expression destination vec-tors,the usefulness of the Gateway cloning system is rather limited for many projects where only a single expression vector is required for a DNA fragment.The two-step cloning process of the Gateway technology is laborious and time consuming for the production of a1This work was supported by the National Science Foundation-Plant Genome Research Program(grant no.0605017).*Corresponding author;e-mail wang.620@.The author responsible for distribution of materials integral to the findings presented in this article in accordance with the policy described in the Instructions for Authors()is: Guo-Liang Wang(wang.620@).[C]Somefigures in this article are displayed in color online but in black and white in the print edition.[W]The online version of this article contains Web-only data.[OA]Open access articles can be viewed online without a sub-scription./cgi/doi/10.1104/pp.109.137125single expression vector.This is particularly true when a large number of plasmids must be cloned.Although a one-step recombinational cloning method was de-scribed to eliminate the production of an entry clone (Fu et al.,2008),the approach is rather limited in scope because long primers containing the specific attach-ment site(att)and two-step PCR are required(Fu et al., 2008).TA cloning is routinely used for cloning of PCR-amplified fragments.This technique exploits the ter-minal transferase activity of some DNA polymerases that add a3#-A overhang to each end of the PCR product.PCR products can be easily cloned into a linearized vector with3#-T overhangs compatible with 3#-A overhangs.Because it is difficult to generate a high-quality TA cloning vector in individual laborato-ries,many TA cloning kits are available in the market. Many of them use blue/white screening for recombi-nants,and the DNA fragments can only be cloned into the TA vector provided in the kit.To meet the need for high-throughput cloning of DNA fragments into di-verse expression vectors,we have developed a signif-icantly improved TA cloning vector system by taking advantage of the negative selection gene marker ccdB to eliminate the self-ligation background after trans-formation.We refer to this new method as the zero background TA cloning system(ZeBaTA).Numerous cloning tests in our laboratory have shown that ZeBaTA provides very high cloning efficiency with almost no self-ligation.Moreover,the ZeBaTA technology can be flexibly adapted for developing specialized expression vectors allowing single-step assembly of PCR-ampli-fied genes or fragments.We demonstrate the feasibility andflexibility of the technology by developing a set of 12transient and12stable transformation vectors for constitutive gene expression,gene silencing,protein tagging,protein subcellular localization,and promoter fragment activity analysis for rice(Oryza sativa)and Arabidopsis(Arabidopsis thaliana).Our results suggest that ZeBaTA technology can also be easily used to develop expression vectors for other organisms(e.g. Escherichia coli,yeast[Saccharomyces cerevisiae],insect, and mammal),thereby providing a novel and general high-throughput platform for functional genomics of target genes.RESULTSConstruction of the ZeBaTA SystemTwo different strategies were used to produce T-vectors,i.e.adding a single thymidine at the3# blunt ends of a linearized vector(Holton and Graham, 1991;Marchuk et al.,1991)and generating single3#-T overhangs of a linearized vector by restriction endo-nuclease digestion(Kovalic et al.,1991;Mead et al., 1991;Ichihara and Kurosawa,1993;Chen et al.,2006a). Although the former has been used to produce com-mercial cloning kits like the pGEM-T system,we selected the restriction endonuclease digestion-mediated strategy to develop a TA cloning vector system because this approach is easy to use for indi-vidual laboratories.Previous publications have de-scribed the use of restriction enzyme Xcm I(Kovalic et al.,1991;Mead et al.,1991)or Ahd I/Eam1105I (Ichihara and Kurosawa,1993;Chen et al.,2006a)to produce intermediate T-vectors.We chose Xcm I as the digestion enzyme to develop the ZeBaTA cloning system because it had a better digestion efficiency than AhdI.Figure1.Construction of the ZeBaTA system.A,Schematic represen-tation of direct cloning of PCR product using the ZeBaTA vector system. The linker of the vector(in gray)is removed after Xcm I digestion yielding a linearized T-vector.B,TA cloning tests of the ZeBaTA system.(1)Self-ligation of Xcm I-digested pGXT using T4DNA ligase from Promega.(2)Ligation of Xcm I-digested pGXT with the PCR product of the rice blast fungus M.oryzae gene MGG_07986.5using T4DNA ligase from Promega.(3)Ligation of Xcm I-digested pGXT with the PCR product of MGG_07986.5using T4DNA ligase from USB Corporation. C,Samples of restriction digestion analysis of the randomly selected colonies derived from ligation of Xcm I-digested pGXT with the PCR product of MGG_07986.5using T4DNA ligase from Promega.pGXT contains two Bam HI recognition sites outside the two Xcm I recognition sites(Supplemental Fig.S1),and MGG_07986.5contains one internal Bam HI site.All samples(lanes1–20)digested by Bam HI released two bands as expected.M,1-kb DNA ladder.Chen et al.The schematic illustration of the improved T-vector system for PCR-amplified gene/fragment cloning is shown in Figure 1A.A pair of Xcm I recognition sites,CCAATACT/TGTATGG,was introduced in the vec-tors,which allowed the generation of a single thymi-dine residue at both 3#ends of the vector when digested with Xcm I.To eliminate the potential self-ligation due to incomplete Xcm I digestion of the vec-tor,the ccdB gene (Bernard and Couturier,1992;Miki et al.,1992),which inhibits growth of E .coli strains by expressing a protein to interfere with its DNA gyrase,was introduced between the two Xcm I sites.Hence,any self-ligation transformants containing the ccdB gene will be eliminated.To test the cloning efficiency of the T-vector system,an intermediate vector pGXT was generated based on the backbone of the pGEM-T easy vector.After Xcm I digestion,ligation reactions of the resulting T-vector alone and T-vector with the PCR-amplified product of the rice blast fungus Mag-naporthe oryzae gene MGG_07986.5were set up follow-ing the standard protocol of the Promega pGEM-T easy vector system.Transformation tests showed that ligation of the T-vector with the MGG_07986.5frag-ments yielded a large number of colonies,whereas ligation of the T-vector alone yielded only a few colonies (Fig.1B).Restriction digestion screening con-firmed that the plasmids yielded from ligation of theT-vector with the PCR product were true recombinants (Fig.1C).To establish a general guide for consistently successful cloning,several factors,such as Xcm I over-digestion for generating a T-vector,insert-to-vector molar ratios,and different T4DNA ligases,were tested to determine their effect on cloning efficiency.Surprisingly,we observed that T4DNA ligases could have a significant impact on cloning efficiency.Liga-tions using Promega T4DNA ligase,the same product used by the pGEM-T easy vector system,consistently gave very high cloning efficiency with almost no self-ligation background.However,regular T4DNA li-gases from USB Corporation usually gave very low ligation efficiency for this TA cloning system (Fig.1B).Although the ligation efficiencies were a little higher at insert-to-vector molar ratios of 4:1to 8:1with the T-vector generated by standard digestion,ligations from vectors with 10-or 20-fold overdigestion and ligations with insert-to-vector molar ratios of 1:1,4:1,8:1,and 12:1all yielded good cloning efficiency when Promega T4DNA ligase was used (data not shown).Set of Expression ZeBaTA Vectors for PlantsUsing ZeBaTA,we developed a set of transient and stable expression vectors for different applications in both dicot and monocot plants.The backbone ofallFigure 2.Site-specific mutagenesis of the maize ubiqutin-1promoter (A)and the backbone of the binary vector pCAMBIA1300(B)in which three Xcm I recognition sites were deleted.The nucleotides represented in lowercase italic letters are the positions where deletions or mutations were made.Kan,Kanamycin resistance gene;LB,T-DNA left border;RB,T-DNA right border.C,Comparison of the levels of GUS expression mediated by the original and modified maize ubiquitin-1promoter in transiently transfected rice protoplasts.GUS activities are represented as a ratio of relative GUS/LUC.The experiment was repeated three times with similar results.1,Protoplast sample transfected with pUbiGUS;2,protoplast sample transfected with pXUN-GUS.A Zero Background Vector Systemtransient expression vectors is derived from pBlue-script II KS (),a high-copy-number cloning vector that can facilitate the isolation of a large amount of plasmid DNA for transient ex-pression.The backbone of all stable expression vectors is derived from pCAMBIA1300(),an Agrobacterium tumefaciens binary vector widely used for transformation in both dicot and monocot plants.Two different promoters,a cauliflower mosaic virus 35S promoter (Odell et al.,1985)and a maize (Zea mays )ubiquitin-1promoter (Christensen et al.,1992)were used to drive expression of genes of interest in dicots and monocots,respectively.The 35S promoter is more efficient in dicots,whereas the maizeubiquitin-1Figure 3.ZeBaTA-based expression vectors for gene overexpression/silencing,protein tagging,protein subcellular localization,and promoter analysis in plants.A,Schematic structures of the transient expression vectors generated by Xcm I digestion.B,Schematic structures of the Agrobacterium -mediated stable transformation vectors generated by Xcm I digestion.LB,T-DNA left border;RB,T-DNA right border.Chen et al.promoter is more efficient in monocots(Christensen et al.,1992).The original maize ubiquitin-1promoter and pCAMBIA1300vector,however,contain one and three Xcm I recognition sites,respectively(Fig.2,A and B).To facilitate the construction of the ZeBaTA-based expression vectors,the Xcm I recognition sites of the maize ubiquitin-1promoter and pCAMBIA1300vec-tor were eliminated by site-specific deletion or site-specific mutation(Fig.2,A and C).The designed expression vectors were all engineered with the cas-sette of the Xcm I-ccdB-Xcm I fragment(Supplemental Fig.S1).Figure3,A and B,illustrates the structural maps of the12transient and12stable transformation T-vectors.All vectors have been tested for cloning at least one time,and the results showed that these ZeBaTA expression vectors,including those binary vectors that are relatively large in size(.10kb), consistently yielded high cloning efficiency(Supple-mental Fig.S2).Because the maize ubiquitin-1promoter used in this system was modified to block its original Xcm I recog-nition site by deleting a single base(Fig.2A)at the position of nucleotide2480,a gus gene(Jefferson et al., 1987)was amplified by PCR and then cloned into the Xcm I-digested pXUN vector to produce an expression construct to test the expression activity of the modified maize ubiquitin-1promoter.The derived constructpXUN-GUS and a control construct pUbi-GUS(Chen et al.,2006b),of which a gus gene is driven by the original maize ubiquitin-1promoter,were tested tran-siently in the transfected rice protoplasts.Transient expression assays showed that the levels of GUS activity in rice protoplasts transfected with these two constructs were similar(Fig.2C),indicating that the deletion of nucleotide2480does not affect the activity of the maize ubiquitin-1promoter.Testing of Tagged Protein ExpressionEpitope tagging is a widely used method for the rapid and effective characterization,purification,and in vivo localization of the protein products of cloned genes.To facilitate gene cloning for epitope tagging in plants,a total of12epitope-tagging vectors(Fig.3,A and B)were constructed using ZeBaTA.These vectors contain a35S promoter or a maize ubiquitin-1pro-moter,allowing direct cloning of genes of interest into expression vectors to express a translational fusion of target protein with three commonly used epitope tags in plants(i.e.FLAG,HA,or Myc;Earley et al.,2006). To determine the feasibility of this epitope-tagging system,a gfp gene was cloned into the pXUN-HA vector to fuse with the HA tag.The resulting expres-sion construct pXUN-HA-GFP was transiently ex-pressed in rice protoplasts.As shown in Figure4,A and B,protoplasts transfected with pXUN-HA-GFP showed strong GFPfluorescence,and HA-tagged GFP protein was detected in protein extracts of trans-fected protoplasts but not in the nontransfected con-trol,demonstrating the potential application of this system for functional study of target proteins in plants.Gene Silencing by Hairpin RNAi or Artificial MicroRNA In plants,a typical and efficient approach to induce gene silencing is to use an inverted-repeat construct to express hairpin RNA(hpRNA;Waterhouse et al.,1998; Smith et al.,2000).However,a major limitation of the hpRNA interference(hpRNAi)approach for high-throughput gene functional analysis is the cumber-some cloning procedure for generating hpRNAi constructs(Helliwell and Waterhouse,2003).The gen-eration of a hpRNAi construct using conventional restriction enzyme digestion and DNA ligation meth-ods usually requires several cloning steps.Although Gateway cloning technology has been adapted to gen-erate hpRNAi constructs(Helliwell and Waterhouse, 2003;Miki and Shimamoto,2004),it still requires two cloning steps.With the ZeBaTA system,hpRNAi con-structs can be made by a single-step cloning procedure (Fig.5A).Instead of making an inverted-repeat cas-sette by DNA recombination techniques,we designed a new approach to assemble the hpRNAi cassette by overlapping PCR.Briefly,a target fragment with an additional3#-terminal sequence complementary to both the5#-and3#-terminal ends of a designed spacer fragment is amplified as afirst step.The overlapping fragments are then fused together in a subsequent PCR reaction,and the resulting inverted-repeat is cloned directly into a ZeBaTA expression vector(Fig.5A).To test the feasibility of this approach,an RNAiconstruct Figure4.Transient expression and protein-tagging detection of the ZeBaTA vectors in rice protoplasts.A,Fluorescence microscopy of the expression of HA-tagged GFP in rice protoplasts.B,Detection of HA-tagged GFP by western ne1,Nontransfected control protoplast sample;lanes2to4,independent protoplast samples transfected with pXUN-HA-GFP.[See online article for color version of thisfigure.]A Zero Background Vector Systemwas generated by overlapping PCR in which the sense and antisense 217-bp fragments of the Arabidopsis phytoene desaturase gene (PDS )were separated by a 420-bp stuffer fragment derived from the gus gene.The resulting fragment was cloned into the pCXSN vector (Fig.3B)to generate the expression construct pCXSN-atPDS-RNAi.The RNAi construct was introduced into Arabidopsis by the floral-dip method.Over 80%of transgenic plants had a clear albino phenotype (Fig.6A),a typical visible phenotype caused by silencing of the PDS gene (Guo et al.,2003;Miki and Shimamoto,2004).Recently,the artificial microRNA (amiRNA)ap-proach has been introduced for highly specific gene silencing in both dicot and monocot plants (Niu et al.,2006;Schwab et al.,2006;Ossowski et al.,2008;Warthmann et al.,2008).Typically,the amiRNA is generated by site-directed mutagenesis on precursors of endogenous miRNAs to exchange the natural miRNA sequences with those of amiRNAs using overlapping PCR (Ossowski et al.,2008).The same ZeBaTA-based vector system developed for ectopic gene expression and hpRNAi can also be used for making amiRNA expression constructs by simpleTAFigure 5.Schematic illustration of the construction of hpRNAi or amiRNA constructs by single-step cloning.A,Generation of hpRNAi constructs by overlapping PCR approach.The target gene fragment and the stuffer sequence fragment are amplified in the first-round PCR.Primers P2,P3,and P4introduce complementary adapters (indicated by vertically lined boxes)to the amplified fragments.The two amplified fragments are fused together as an inverted-repeat cassette in the second-round PCR by using single P1primer.The resulting fragment is then directly cloned into the plant expression T-vector.B,Generation of amiRNA constructs by overlapping PCR approach.C,Generation of amiRNA constructs for rice genes by single-step PCR.The expression vectors pXUN-osaMIR528and pCXUN-osaMIR528were preassembled with 5#and 3#stemloop backbone sequences of a rice miRNA precursor Osa-MIR-528(Warthmann et al.,2008).Thus,making amiRNA constructs for rice target genes only requires an amiRNA-amiRNA*fragment generated from single-step PCR.The nucleotides represented in lowercase letters are the positions where mutations were made to introduce two Xcm I recognition sites.Chen et al.cloning (Fig.5B),thus bypassing the time-consuming two-step procedure for the regular restriction enzyme digestion-mediated cloning or the Gateway cloning (Ossowski et al.,2008).We further developed a ZeBaTA-amiRNA system to simplify the generation of rice amiRNA constructs because our lab is focusing on rice functional genomics.The new ZeBaTA-amiRNA vector was designed based on the stemloop backbone derived from Osa-MIR528,an endogenous rice miRNA precursor that has been used to efficiently express amiRNAs for highly specific silencing of targeted genes in rice (Warthmann et al.,2008).By site-directed muta-genesis of a single base on the 5#and 3#stemloop backbones of Osa-MIR528,respectively,a cassette of 5#Osa-MIR528stemloop backbone-Xcm I-ccdB -Xcm I-3#Osa-MIR528was assembled and cloned into the expres-sion vectors where the expression of amiRNA is under the control of the maize ubiquitin-1promoter.Figure 5C illustrates the structural maps of the Osa-MIR528-based vectors pXUN-osaMIR528and pCXUN-osaMIR528.The vectors allow for high-throughput generationof rice amiRNA constructs by cloning the amiRNA-amiRNA*fragment generated from a single-step PCR into the ZeBaTA vector with the preassembled Osa-MIR528stemloop backbone (Fig.5C;Supplemental Fig.S3),thus avoiding the time-consuming overlapping PCR.The modified vector was evaluated by expression of the amiRNA for silencing of the OsPDS gene.The two constructs pCXUN-amiPDS and pCXUN528-PDS,which contain original or modified Osa-MIR528stem-loop backbone with amiRNA sequence targeting OsPDS ,respectively ,were introduced into rice cv Nip-ponbare by Agrobacterium -mediated transformation.Consistent with a previous study (Warthmann et al.,2008),70.1%of the primary transgenic lines transformed with pCXUN-amiPDS had a bleaching PDS silencing phenotype (Fig.6B;Table I).Similarly,77.1%of the primary transgenic lines transformed with pCXUN528-PDS had the same albino phenotype,suggesting that the mutagenesis on the Osa-MIR528stemloop backbone does not affect the biogenesis of the amiRNA for silenc-ing of the PDS gene.Protein Subcellular Localization/Colocalization and Promoter Activity AssayTo investigate the subcellular localization or colo-calization of particular proteins,a set of ZeBaTA vectors (i.e.pXDG,pXDR,pCXDG,and pCXDR)was devised for transient or stable expression of protein fusions with GFP or red fluorescent protein.The vectors contain a 35S promoter-driven gfp or DsRed cassette that has been used to visualize protein local-ization in both dicot and monocot plants (Goodin et al.,2002;Chen et al.,2006b).As shown in Figure 3,A and B,PCR products of genes of interest can be simply engineered into the vectors to fuse with the gfp or DsRed gene.To confirm whether the vectors can be used for detecting protein localization in plant cells,the rice Spin1gene encoding a putative RNA-binding protein previously shown to be nuclear targeted(Vega-Sa´nchez et al.,2008)was cloned into vectors pXDG and pXDR to fuse in-frame with gfp and DsRed ,respectively.Transient expression of the constructs pXDG-Spin1and pXDR-Spin1in rice protoplasts dem-onstrated that the GFP-and DsRed-SPIN1fusion proteins were targeted to the nuclear region as pre-dicted (Fig.7A).For promoter activity assays,two reporters,gus and gfp ,were used for constructing pXGUS-P/pCXGUS-P and pXGFP-P/pCXGFP-P ,respectively.The linear T-vectors of pXGUS-P/pCXGUS-P orpXGFP-P/Figure 6.Silencing of the PDS gene in Arabidopsis and rice by the ZeBaTA-based hpRNAi or amiRNA approaches.A,Arabidopsis plants transformed with the hpRNAi construct pCXSN-atPDS-RNAi showing the PDS silencing albino phenotype.(1)Control plant;(2and 3)two examples of transgenic Arabidopsis plants.B,Rice plants transformed with the amiRNA vectors showing the albino phenotype.(1)Control plant;(2)example of pCXUN-amiPDS-transformed plants;and (3)example of pCXUN528-PDS-transformed plants.C,RT-PCR analysis of PDS suppression transgenic rice plants.Five independent primary plants (1,2,3,4,and 5)transformed with pCXUN-amiPDS and five independent primary plants (6,7,8,9,and 10)transformed with pCXUN528-PDS were selected for the analysis.CK,Wild-type Nip-ponbare plant used as the control.Table I.PDS silencing frequency of transgenic rice mediated by the ZeBaTA-amiRNA systemamiRNA VectorTotal Independent TransformantsAlbino PhenotypeEfficiency%pCXUN-amiPDS 553970.1pCXUN528-PDS 352777.1A Zero Background Vector SystempCXGFP-P (Fig.3,A and B)allow direct cloning of PCR-amplified promoter fragments located in front of the reporter genes.As proof of concept,the 35S pro-moter was cloned into pCXGUS-P to drive expression of the reporter gene gus .Arabidopsis plants stably transformed with the construct pCX-35S-GUS showed constitutive GUS expression in the whole plants (Fig.7B),confirming the feasibility of the system for assay-ing promoter activity.DISCUSSIONWith the rapid development of the next-generation sequencing technology,more plant genomes will be sequenced in the near future.How to rapidly deter-mine the function of the identified genes on a large scale is a daunting challenge.The ability to efficiently make constructs to transiently and stably express specific genes in cells,tissues,or whole plants is a fundamental aspect and bottle neck of plant functional genomics research.Traditionally,the cloning vectorsfor plant research carry a multicloning site (MCS)within their target gene expression cassettes.The restriction sites in the MCS are rather limited,making cloning of most target genes difficult.Although TA cloning vectors have been widely used for cloning of PCR-amplified fragments,the system has not yet been incorporated in the cloning vectors for transient and stable expression of target genes because of the tech-nical challenge of generating low-background TA cloning vectors.The Gateway system has been a popular choice for generating various constructs be-cause it allows the gene of interest to be easily cloned into specifically designed plasmids without DNA re-striction digestions.The two-step cloning and expen-sive reagents,however,make the Gateway system impractical for large-scale cloning in most individual laboratories when the entry clone collections are not available.The ZeBaTA system described here over-comes the limitations of both the TA and Gateway cloning systems.After two Xcm I recognition sites have been introduced into the MCS,any PCR fragments with a T-overhang can be easily cloned into a ZeBaTA vector.With the introduction of the negative selection marker gene ccdB between the two Xcm I sites,any self-ligation transformants are ing this tech-nology,we constructed a set of 12transient and 12stable transformation vectors for plant gene expres-sion studies and tested the vectors in rice or Arabi-dopsis in our laboratories.These vectors can be used in a wide range of functional genomics projects in plants and will be distributed to the research community upon request.Under certain conditions,cloning with T-vectors generated by digestion with Ahd I or Xcm I gave low efficiency and the T residue of the insert-vector junc-tion in the recombinant clones is often missing (Mead et al.,1991;Chen et al.,2006a).Chen et al.(2006a)speculated that this may be due to the presence of unknown factors that,during digestion and prepara-tion of the T-vectors,influence the stability of 3#-T overhangs.In this study,we found that the main factor affecting successful cloning is the use of an appropri-ate T4DNA ligase.We tested the Promega T4DNA ligase,which is included in the pGEM-T easy vector system,and the T4DNA ligase from USB Corporation.The ligations using Promega T4DNA ligase consis-tently gave a very high cloning efficiency;most of the ligations using USB Corporation T4DNA ligases yielded low efficiency.Many of the recombinant plas-mids from the latter ligations missed a T residue in the insert-vector junction,consistent with observations by Mead et al.(1991)and Chen et al.(2006a).The T residue is missing mainly because regular commercial T4DNA ligases contain exonuclease activities that can remove the 3#-T tails from the vector,as reported in the technical manual of the pGEM-T and pGEM-T easy vector systems (/tbs/tm042/tm042.pdf);removal of the 3#-T tails from the vector results in very low cloning efficiency.When the Promega T4DNA ligase was used for ligation,weFigure 7.Protein subcellular localization and promoter activity anal-ysis using the ZeBaTA vectors.A,Fluorescence microscopy of the coexpression of GFP and DsRed,or GFP-SPIN1and DsRed-SPIN1fusions in rice protoplasts.Scale bar =20m m.The RNA binding nuclearprotein SPIN1was used as a tester (Vega-Sa´nchez et al.,2008).B,GUS staining of Arabidopsis transformed with pCX-35S-GUS,where the 35S promoter was cloned into the vector pCXGUS-P to test the system.CK,Plant transformed with control vector pCAMBIA1300();pCX-35S-GUS-1and pCX-35S-GUS-2,two independent primary transgenic plants.Chen et al.。
克隆的英语作文
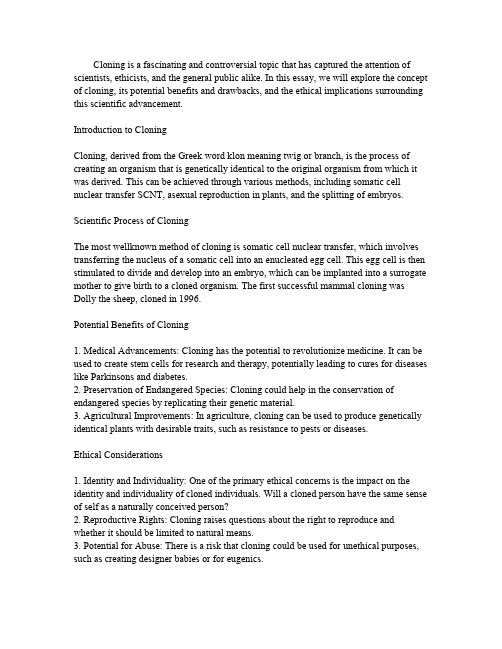
Cloning is a fascinating and controversial topic that has captured the attention of scientists,ethicists,and the general public alike.In this essay,we will explore the concept of cloning,its potential benefits and drawbacks,and the ethical implications surrounding this scientific advancement.Introduction to CloningCloning,derived from the Greek word klon meaning twig or branch,is the process of creating an organism that is genetically identical to the original organism from which it was derived.This can be achieved through various methods,including somatic cell nuclear transfer SCNT,asexual reproduction in plants,and the splitting of embryos.Scientific Process of CloningThe most wellknown method of cloning is somatic cell nuclear transfer,which involves transferring the nucleus of a somatic cell into an enucleated egg cell.This egg cell is then stimulated to divide and develop into an embryo,which can be implanted into a surrogate mother to give birth to a cloned organism.The first successful mammal cloning was Dolly the sheep,cloned in1996.Potential Benefits of Cloning1.Medical Advancements:Cloning has the potential to revolutionize medicine.It can be used to create stem cells for research and therapy,potentially leading to cures for diseases like Parkinsons and diabetes.2.Preservation of Endangered Species:Cloning could help in the conservation of endangered species by replicating their genetic material.3.Agricultural Improvements:In agriculture,cloning can be used to produce genetically identical plants with desirable traits,such as resistance to pests or diseases.Ethical Considerations1.Identity and Individuality:One of the primary ethical concerns is the impact on the identity and individuality of cloned individuals.Will a cloned person have the same sense of self as a naturally conceived person?2.Reproductive Rights:Cloning raises questions about the right to reproduce and whether it should be limited to natural means.3.Potential for Abuse:There is a risk that cloning could be used for unethical purposes, such as creating designer babies or for eugenics.Legal and Regulatory IssuesMany countries have enacted laws to regulate or ban human cloning due to the ethical concerns it raises.However,the regulation of animal cloning is less uniform,with some countries allowing it for research and agricultural purposes.ConclusionCloning is a complex scientific process with the potential to bring about significant benefits in various fields.However,it also presents a myriad of ethical and legal challenges that society must carefully consider.As our understanding of genetics and cloning technology advances,it is crucial to engage in ongoing discussions about the implications of these developments for individuals,society,and the environment.。
分子生物学名词解释大全

分子生物学名词解释大全AAbundance (mRNA 丰度):指每个细胞中mRNA 分子的数目。
Abundant mRNA(高丰度mRNA):由少量不同种类mRNA组成,每一种在细胞中出现大量拷贝。
Acceptor splicing site (受体剪切位点):内含子右末端和相邻外显子左末端的边界。
Acentric fragment(无着丝粒片段):(由打断产生的)染色体无着丝粒片段缺少中心粒,从而在细胞分化中被丢失。
Active site(活性位点):蛋白质上一个底物结合的有限区域。
Allele(等位基因):在染色体上占据给定位点基因的不同形式。
Allelic exclusion(等位基因排斥):形容在特殊淋巴细胞中只有一个等位基因来表达编码的免疫球蛋白质。
Allosteric control(别构调控):指蛋白质一个位点上的反应能够影响另一个位点活性的能力。
Alu-equivalent family(Alu 相当序列基因):哺乳动物基因组上一组序列,它们与人类Alu家族相关。
Alu family (Alu家族):人类基因组中一系列分散的相关序列,每个约300bp长。
每个成员其两端有Alu 切割位点(名字的由来)。
α-Amanitin(鹅膏覃碱):是来自毒蘑菇Amanita phalloides 二环八肽,能抑制真核RNA聚合酶,特别是聚合酶II 转录。
Amber codon (琥珀密码子):核苷酸三联体UAG,引起蛋白质合成终止的三个密码子之一。
Amber mutation (琥珀突变):指代表蛋白质中氨基酸密码子占据的位点上突变成琥珀密码子的任何DNA 改变。
Amber suppressors (琥珀抑制子):编码tRNA的基因突变使其反密码子被改变,从而能识别UAG 密码子和之前的密码子。
Aminoacyl-tRNA (氨酰-tRNA):是携带氨基酸的转运RNA,共价连接位在氨基酸的NH2基团和tRNA 终止碱基的3¢或者2¢-OH 基团上。
小分子 RNA
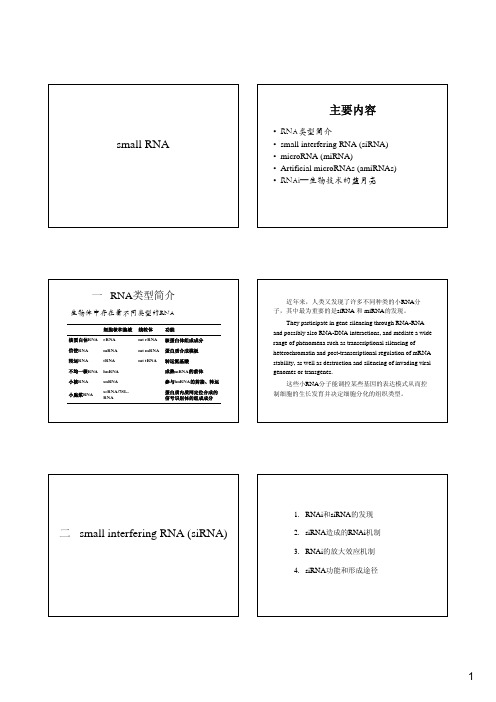
Figure 1. Comparison of small RNA pathways mediating transgene-induced gene silencing in plants.Long dsRNAs derived from inverted repeat constructs (hpRNAi, VIGS) or generated from ssRNAof the RDR family (co-suppression, VIGS) are processed by DCL2, DCL3 or DCL4 to 22-, 24-or 21-nucleotide siRNAs, respectively. 21-nucleotide siRNAs guide cleavage of target transcripts by AGO1, while 24-nucleotide guide AGO4-mediated chromosome modification involving proteins with chromatin-modifying activities (CMAs). Each DCL protein interacts with a specific dsRNA binding protein, DRB. siRNA duplexes are 2’methylated at the 3’end by HEN1. prom, promoter; vRDR, gene for viral RDR. Black bars correspond to•overexpression ) The) Plants expressing miR159-resistant MYB33 (b) Plants expressing miR166-resistant PHB (c) miR160-resistant ARF17 seedlings have up to four cotyledons, with a leaf emerging between each pair of cotyledons (d) flowers expressing a miR164-resistant CUC1often display two sepals (top) and six petals (bottom). (e) miR172-resistant AP2 transgenic flowerstranscripts and mutation of one orsensitivity and specificity criteria.design (Oligo). bin/mirnatools.pl?page=1A. Input sequences4. Procedure -amiRNA design step by stepB. OutputYour email willcontain a link to your result page.Result page of WMD2 amiRNA Designer: amiRNAs are sortedaccording to established quality criteria. AmiRNAs at the top of the list are expected to work better in most cases.•Sequence features of endogenous and artificial miRNAs.(a) Sequence biases of conserved endogenous miRNAs from Arabidopsis thaliana, poplar and rice miRNAs (only non-identical members of conserved families in Rfam are considered). (b) Observed mismatch frequency betweenendogenous miRNAs and their validated targets compared with design principles for amiRNAs. MM, mismatches.•target searchTo facilitate theselection of amiRNAs, their sequences are hyperlinked to an alignment to all transcripts in the respective genomerelease that have up to 5 mismatches. They are sorted by hybridization energy between target gene and amiRNA, and your intended target gene(s) should appear on top of the list.•Search resultsD. Oligo design Once selected, the amiRNA sequence needs to be engineered into an endogenous microRNA precursor for expression. We provide the "Oligo" tool, which designs primer for the Arabidopsis thaliana MIR319a (ath-miR319a) and Oryza sativa MIR528 (osa-miR528) precursors. You simply have to paste your amiRNA sequence and retrieve 4 oligo sequences (they are in 5'->3' orientation already),Suppression of CXCR4 by short-interfering RNA.HeLa-green fluorescent protein (GFP) cells were mock transfected (control) or transfected interfering RNA (siRNA) directed to CXCR4 (RNA X42i ). After 48 h, cells were with anti-CXCR4 monoclonal antibody (12G5) and a secondaryrhodamine-conjugated antibody and visualized in a Nikon fluorescence microscope. CXCR4 and GFP expression were quantified by flow cytometry analysis. Mean。
MicroRNAs与异种移植
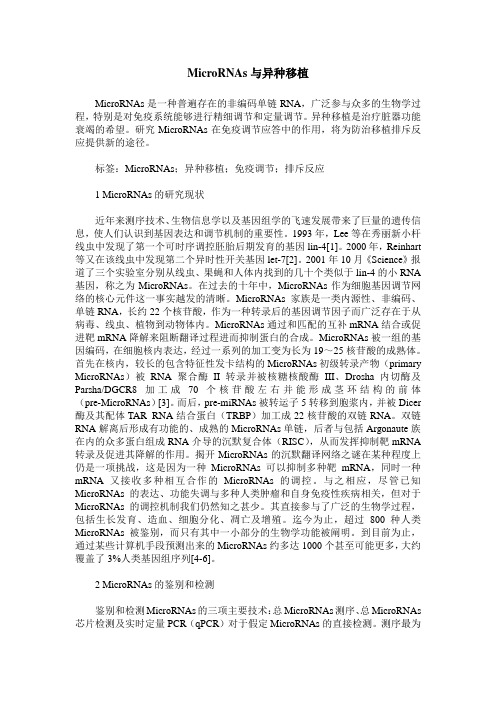
MicroRNAs与异种移植MicroRNAs是一种普遍存在的非编码单链RNA,广泛参与众多的生物学过程,特别是对免疫系统能够进行精细调节和定量调节。
异种移植是治疗脏器功能衰竭的希望。
研究MicroRNAs在免疫调节应答中的作用,将为防治移植排斥反应提供新的途径。
标签:MicroRNAs;异种移植;免疫调节;排斥反应1 MicroRNAs的研究现状近年来测序技术、生物信息学以及基因组学的飞速发展带来了巨量的遗传信息,使人们认识到基因表达和调节机制的重要性。
1993年,Lee等在秀丽新小杆线虫中发现了第一个可时序调控胚胎后期发育的基因lin-4[1]。
2000年,Reinhart 等又在该线虫中发现第二个异时性开关基因let-7[2]。
2001年10月《Science》报道了三个实验室分别从线虫、果蝇和人体内找到的几十个类似于lin-4的小RNA 基因,称之为MicroRNAs。
在过去的十年中,MicroRNAs作为细胞基因调节网络的核心元件这一事实越发的清晰。
MicroRNAs家族是一类内源性、非编码、单链RNA,长约22个核苷酸,作为一种转录后的基因调节因子而广泛存在于从病毒、线虫、植物到动物体内。
MicroRNAs通过和匹配的互补mRNA结合或促进靶mRNA降解来阻断翻译过程进而抑制蛋白的合成。
MicroRNAs被一组的基因编码,在细胞核内表达,经过一系列的加工变为长为19~25核苷酸的成熟体。
首先在核内,较长的包含特征性发卡结构的MicroRNAs初级转录产物(primary MicroRNAs)被RNA聚合酶II转录并被核糖核酸酶III、Drosha内切酶及Parsha/DGCR8加工成70个核苷酸左右并能形成茎环结构的前体(pre-MicroRNAs)[3]。
而后,pre-miRNAs被转运子5转移到胞浆内,并被Dicer 酶及其配体TAR RNA结合蛋白(TRBP)加工成22核苷酸的双链RNA。
克隆技术 英语作文

克隆技术英语作文Cloning Technology。
Cloning technology, also known as somatic cell nuclear transfer, is a revolutionary scientific advancement that has the potential to change the world as we know it. The process involves taking the nucleus of a somatic cell from an organism and inserting it into an egg cell that has had its nucleus removed. The resulting embryo is then implanted into a surrogate mother, where it develops into a genetically identical copy of the original organism.The potential applications of cloning technology are vast and varied. In the field of medicine, it holds the promise of providing a limitless supply of organs and tissues for transplantation, as well as the ability to create custom-made stem cells for the treatment of a wide range of diseases and injuries. In agriculture, it could be used to produce genetically superior livestock and crops, leading to increased food production and improved foodsecurity. In conservation, it offers the possibility of preserving endangered species and restoring ecosystems that have been damaged by human activity.Despite its potential benefits, cloning technology is not without its controversies and ethical concerns. One of the most contentious issues is the prospect of human cloning, which raises questions about the nature ofidentity, individuality, and the sanctity of human life. There are also concerns about the welfare of cloned animals, as well as the potential impact on biodiversity and the environment.In recent years, significant progress has been made in the field of cloning technology. In 1996, the birth ofDolly the sheep, the first mammal to be cloned from anadult somatic cell, captured the world's imagination and sparked a flurry of research and development in the field. Since then, scientists have successfully cloned a widerange of animals, including mice, pigs, and monkeys, andthe technology continues to advance at a rapid pace.Despite the progress that has been made, there arestill many challenges and obstacles that need to be overcome before cloning technology can reach its full potential. One of the biggest challenges is the low success rate of the cloning process, which results in a high rate of miscarriages and abnormalities in cloned animals. There are also technical and logistical challenges associated with scaling up the technology for commercial use, as well as regulatory and ethical considerations that need to be addressed.In conclusion, cloning technology has the potential to revolutionize the fields of medicine, agriculture, and conservation, but it also raises a host of ethical and practical challenges that need to be carefully considered. As the technology continues to advance, it is important for scientists, policymakers, and the public to engage in informed and thoughtful discussions about the potential benefits and risks of cloning technology, and to work together to ensure that it is used in a responsible and ethical manner. Only through careful consideration and responsible use can we fully realize the potential ofcloning technology for the benefit of humanity and the natural world.。
克隆动物的作文六级英语
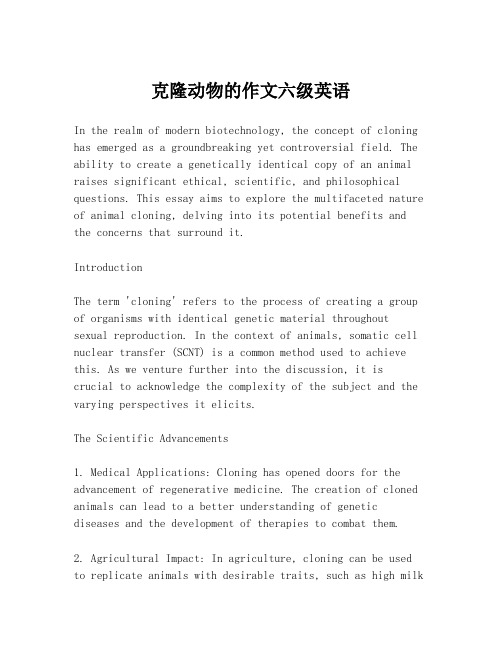
克隆动物的作文六级英语In the realm of modern biotechnology, the concept of cloning has emerged as a groundbreaking yet controversial field. The ability to create a genetically identical copy of an animal raises significant ethical, scientific, and philosophical questions. This essay aims to explore the multifaceted nature of animal cloning, delving into its potential benefits and the concerns that surround it.IntroductionThe term 'cloning' refers to the process of creating a group of organisms with identical genetic material throughout sexual reproduction. In the context of animals, somatic cell nuclear transfer (SCNT) is a common method used to achieve this. As we venture further into the discussion, it iscrucial to acknowledge the complexity of the subject and the varying perspectives it elicits.The Scientific Advancements1. Medical Applications: Cloning has opened doors for the advancement of regenerative medicine. The creation of cloned animals can lead to a better understanding of genetic diseases and the development of therapies to combat them.2. Agricultural Impact: In agriculture, cloning can be used to replicate animals with desirable traits, such as high milkproduction in cows or lean meat in livestock, which can significantly improve farming efficiency.3. Conservation Efforts: For endangered species, cloning presents a potential method to preserve genetic diversity and prevent extinction.Ethical Considerations1. Welfare Concerns: The process of cloning can be fraughtwith health issues for the cloned animals, leading toconcerns about animal welfare and the moral implications of subjecting animals to such procedures.2. Playing God: Some argue that cloning represents anoverstep of human boundaries, suggesting that we areventuring into a realm that is not ours to manipulate.3. Commercialization: The commercial use of cloning, such as for the creation of pets that meet specific consumer demands, raises questions about the commodification of life.The Debate on Animal RightsThe debate on animal rights in the context of cloning is particularly poignant. While some see it as a means to an end, others view it as exploitation. The question of whether animals have inherent rights that should be respected, independent of their utility to humans, is central to this debate.The Legal LandscapeLaws and regulations regarding animal cloning vary widely across different countries. While some have banned the practice entirely, others have more lenient regulations, allowing for research and development under strict ethical guidelines.ConclusionThe cloning of animals is a complex issue that straddles the boundaries of science, ethics, and law. As we continue to unravel the mysteries of genetic replication, it is imperative that we engage in a thoughtful and balanced discourse that respects both the potential of science and the ethical considerations that come with it. The future of cloning will undoubtedly be shaped by the collective wisdom of scientists, ethicists, lawmakers, and society at large.Word Bank for Advanced Vocabulary- Biotechnology: The use of living organisms or their components to make or modify products for specific use.- Somatic cell nuclear transfer (SCNT): A process used in cloning where the nucleus of a somatic cell is transferred to an egg cell.- Regenerative medicine: A branch of medicine that deals with the replacement or regeneration of cells, tissues, or organs to restore impaired function.- Endangered species: Species that are at risk of becoming extinct due to various threats.- Welfare: The state of being well, healthy, and prosperous. - Commodification: The process of turning something into a commodity, especially over time.- Ethical guidelines: A set of principles that provide a basis for moral judgment.- Collective wisdom: The accumulated knowledge, experience, and good judgment of a group of people.This essay is structured to provide a comprehensive overview of the topic, using advanced vocabulary appropriate for a Level 6 English proficiency. It is designed to stimulate critical thinking and encourage further research into the subject matter.。
cloning英文作文

cloning英文作文Title: The Science and Ethics of Cloning。
Cloning, the process of creating genetically identical copies of organisms, has been a topic of intense debate and fascination since its inception. From the cloning of Dolly the sheep in 1996 to recent advancements in cloning technology, the field has made significant strides. However, with these advancements come complex ethical considerations and scientific challenges.Firstly, it's essential to understand the sciencebehind cloning. Cloning typically involves taking the nucleus of a somatic cell from the organism to be clonedand inserting it into an egg cell that has had its nucleus removed. This egg cell, now containing the genetic material of the donor organism, is then stimulated to divide and develop into an embryo, which can be implanted into a surrogate mother and brought to term.One of the primary purposes of cloning is to replicate organisms with desirable traits, whether for agricultural, medical, or conservation purposes. For example, in agriculture, cloning can be used to reproduce high-yielding crops or livestock with desirable genetic traits, potentially increasing food production and sustainability. In medicine, cloning holds promise for producing tissues and organs for transplantation, thereby addressing the shortage of donor organs.However, alongside its potential benefits, cloning raises significant ethical concerns. One of the most pressing issues is the welfare of cloned animals. Studies have shown that cloned animals often suffer from health problems and abnormalities, such as premature aging, organ failure, and immune deficiencies. These issues raise questions about the ethics of subjecting animals to potential suffering in the name of scientific advancement or commercial gain.Moreover, cloning also raises questions about human identity and individuality. If human cloning were to becomea reality, it could challenge our fundamental understanding of what it means to be unique and autonomous individuals.It could also raise concerns about the exploitation of cloned individuals or the potential for genetic discrimination.Another ethical consideration is the potential misuse of cloning technology. Cloning could be used for nefarious purposes, such as cloning humans for reproductive purposes or creating clones for exploitation or experimentation. The prospect of human cloning, in particular, raises profound moral and existential questions about the sanctity of life and the limits of scientific intervention.In addition to ethical concerns, cloning also presents scientific challenges and limitations. Despite advancements in cloning technology, the process remains inefficient and prone to errors. Many cloned embryos fail to develop properly, and those that do often suffer from health problems. These limitations make cloning a risky and unpredictable endeavor, raising doubts about itspracticality and feasibility in various contexts.Furthermore, cloning raises ecological concerns, particularly regarding the conservation of endangered species. While cloning could theoretically be used to restore extinct species or bolster dwindling populations,it raises questions about the integrity of natural ecosystems and the potential unintended consequences of reintroducing cloned organisms into the wild.In conclusion, cloning is a complex and multifaceted issue that intersects science, ethics, and society. Whileit holds promise for various applications, including agriculture, medicine, and conservation, it also raises profound ethical concerns and scientific challenges. As the field of cloning continues to advance, it is essential to approach it with careful consideration of its potential benefits and risks, as well as its broader implications for humanity and the natural world.。
英语作文 克隆
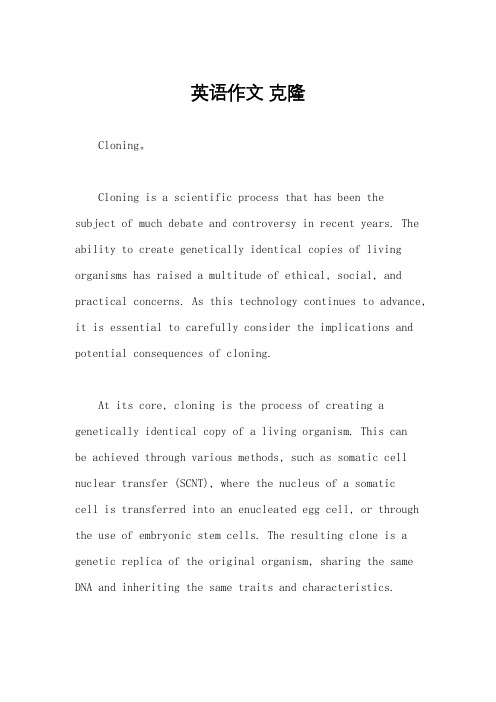
英语作文克隆Cloning。
Cloning is a scientific process that has been thesubject of much debate and controversy in recent years. The ability to create genetically identical copies of living organisms has raised a multitude of ethical, social, and practical concerns. As this technology continues to advance, it is essential to carefully consider the implications and potential consequences of cloning.At its core, cloning is the process of creating a genetically identical copy of a living organism. This canbe achieved through various methods, such as somatic cell nuclear transfer (SCNT), where the nucleus of a somaticcell is transferred into an enucleated egg cell, or through the use of embryonic stem cells. The resulting clone is a genetic replica of the original organism, sharing the same DNA and inheriting the same traits and characteristics.One of the primary applications of cloning is in the field of medicine and scientific research. Cloning can be used to create genetically identical animal models for the study of diseases, the testing of new drugs, and the development of personalized therapies. By having access to cloned organisms, researchers can gain valuable insights into the underlying mechanisms of various health conditions and explore potential treatments with greater precision and accuracy.Additionally, cloning has been proposed as a means of preserving endangered species or reviving extinct ones. The ability to clone rare or extinct animals could potentially help to restore depleted populations and safeguard the biodiversity of our planet. However, the ethical and practical challenges of such endeavors remain a subject of ongoing debate.Despite the potential benefits of cloning, there are significant ethical concerns that must be addressed. One of the primary concerns is the issue of human cloning, which has been the subject of intense scrutiny and controversy.The prospect of creating genetically identical human beings raises profound questions about the sanctity of human life, the potential for exploitation, and the societal implications of such a technology.Another ethical concern is the potential for cloning to be used for reproductive purposes, where individuals or couples may seek to create a genetic copy of themselves or a loved one. This raises complex questions about the rights and welfare of the clone, as well as the potential for the creation of a "designer baby" industry, where individuals could selectively choose the genetic traits of their offspring.Furthermore, the use of cloning technology in agricultural and livestock production has also raised concerns about the potential impact on food security, animal welfare, and the environment. The widespread adoption of cloned animals in the food supply chain could lead to a reduction in genetic diversity, which could make the entire system more vulnerable to disease outbreaks or environmental changes.In addition to the ethical considerations, there are also practical and technical challenges associated with cloning. The process of cloning is often inefficient, with low success rates and a high incidence of developmental abnormalities in the resulting clones. This can lead to significant financial and emotional costs, as well as potential risks to the health and wellbeing of the cloned organisms.As the field of cloning continues to evolve, it is essential that policymakers, scientists, and the general public engage in a robust and ongoing dialogue to address the complex issues surrounding this technology. This dialogue should consider the potential benefits and risks, the ethical implications, and the appropriate regulatory frameworks to ensure that cloning is developed and utilized in a responsible and ethical manner.In conclusion, cloning is a powerful and potentially transformative technology that holds both promise and peril. While the potential benefits of cloning in the fields ofmedicine, conservation, and scientific research are significant, the ethical and practical challenges must be carefully navigated. As we move forward, it is crucial that we approach this technology with a deep sense of responsibility, a commitment to ethical principles, and a dedication to the well-being of all living beings.。
- 1、下载文档前请自行甄别文档内容的完整性,平台不提供额外的编辑、内容补充、找答案等附加服务。
- 2、"仅部分预览"的文档,不可在线预览部分如存在完整性等问题,可反馈申请退款(可完整预览的文档不适用该条件!)。
- 3、如文档侵犯您的权益,请联系客服反馈,我们会尽快为您处理(人工客服工作时间:9:00-18:30)。
Cloning of artificial microRNAs
Protocol by Rebecca Schwab MPI for Developmental Biology
Tuebingen, 2005
The artificial microRNA designer WMD delivers 4 oligonucleotide sequences (I to IV), which are used to engineer your artificial microRNA into the endogenous miR319a precursor by site-directed mutagenesis.
As a template for the PCRs, you need the plasmid pRS300, which contains the miR319a precursor in pBSK (cloned via SmaI site).
To request this plasmid, please send an email to Detlef Weigel (weigel@).
map of pRS300:
sequence of pRS300:
Cloning strategy:
I: microRNA forward
II: microRNA reverse
III: microRNA* forward
IV: microRNA* reverse
The amiRNA containing precursor is generated by overlapping PCR. A first round amplifies fragments (a) to (c), which are listed in the table above. These are subsequently fused in PCR (d).
Oligonucleotides A and B are based on the template plasmid sequence. They are located outside of the multiple cloning site of pBSK to generate bigger PCR products.
Their sequences:
A 5’ CTG CAA GGC GAT TAA GTT GGG TAA C 3’
B 5’ GCG GAT AA
C AAT TTC ACA CAG GAA ACA G 3’
Cloning protocol:
PCR reactions (a), (b), (c):
5µl 10xPCR buffer (with Mg++)
5µl dNTPs @ 2mM
2µl each oligo @10µM
2µl plasmid DNA (1:100) 0.5µl Pfu
33.5µl water
------------
-> 50µl 95°C 2’
95°C 30”
55°C 30” -> lower temp. for (b) 72°C 40”
-> 24 cycles
72°C 7’
run on 2% gel -> cut bands -> elute in 20µl water PCR reaction (d):
5µl 10xPCR buffer (with Mg++)
5µl dNTPs @ 2mM 2µl oligo A @ 10µM 2µl oligo B @ 10µM 0.5µl PCR (a)
0.5µl PCR (b)
0.5µl PCR (c)
0.5µl Pfu
34.5µl water
-------------
-> 50µl 95°C 2’95°C 30”55°C 30”72°C 1’30”-> 24 cycles 72°C 7’
run on 1% gel -> cut bands -> elute in 20µl water
Cloning of PCR products:
Any vector possible, but make sure that you choose the right sites to sequence. T3 and T7 are already part of the PCR fragment.
A-tailing of PCR products and cloning in to pGEM-T-easy works reliably, sequencing can be carried out with oligonucleotides A and B.
To release the amiRNA precursor fragment, any sites of the pBSK multiple cloning site can be used, since they are part of the PCR fragment. We normally use the EcoRI and BamHI sites. EcoRI is at the 5’, BamHI at the 3’ end of the precursor.
Alternative PCR strategy:。