Spectroscopic Dating of Stellar Populations from Local Star Clusters to Distant Galaxies an
A Spectroscopic Search for the non-nuclear Wolf-Rayet Population of the metal-rich spiral g
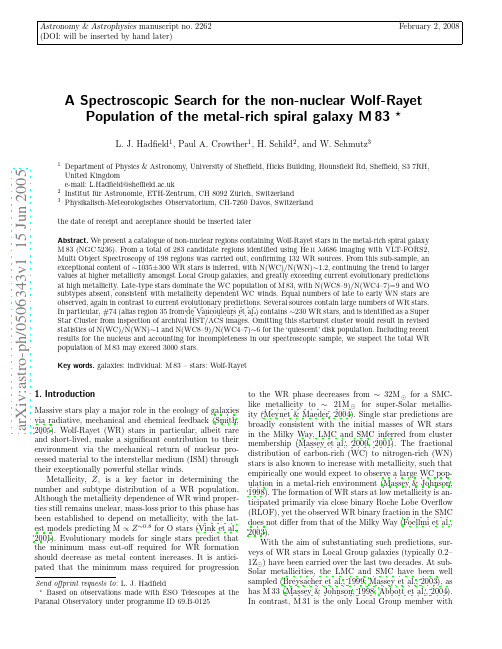
a r X i v :a s t r o -p h /0506343v 1 15 J u n 2005Astronomy &Astrophysics manuscript no.2262February 2,2008(DOI:will be inserted by hand later)A Spectroscopic Search for the non-nuclear Wolf-Rayet Population of the metal-rich spiral galaxy M 83⋆L.J.Hadfield 1,Paul A.Crowther 1,H.Schild 2,and W.Schmutz 31Department of Physics &Astronomy,University of Sheffield,Hicks Building,Hounsfield Rd,Sheffield,S37RH,United Kingdome-mail:L .Hadfield@sheffi 2Institut f¨u r Astronomie,ETH-Zentrum,CH 8092Z¨u rich,Switzerland3Physikalisch-Meteorologisches Observatorium,CH-7260Davos,Switzerlandthe date of receipt and acceptance should be inserted laterAbstract.We present a catalogue of non-nuclear regions containing Wolf-Rayet stars in the metal-rich spiral galaxy M 83(NGC 5236).From a total of 283candidate regions identified using He ii λ4686imaging with VLT-FORS2,Multi Object Spectroscopy of 198regions was carried out,confirming 132WR sources.From this sub-sample,an exceptional content of ∼1035±300WR stars is inferred,with N(WC)/N(WN)∼1.2,continuing the trend to larger values at higher metallicity amongst Local Group galaxies,and greatly exceeding current evolutionary predictions at high te-type stars dominate the WC population of M 83,with N(WC8–9)/N(WC4–7)=9and WO subtypes absent,consistent with metallicity dependent WC winds.Equal numbers of late to early WN stars are observed,again in contrast to current evolutionary predictions.Several sources contain large numbers of WR stars.In particular,#74(alias region 35from de Vaucouleurs et al.)contains ∼230WR stars,and is identified as a Super Star Cluster from inspection of archival HST/ACS images.Omitting this starburst cluster would result in revised statistics of N(WC)/N(WN)∼1and N(WC8–9)/N(WC4–7)∼6for the ‘quiescent’disk population.Including recent results for the nucleus and accounting for incompleteness in our spectroscopic sample,we suspect the total WR population of M 83may exceed 3000stars.Key words.galaxies:individual:M 83–stars:Wolf-Rayet1.IntroductionMassive stars play a major role in the ecology of galaxies via radiative,mechanical and chemical feedback (Smith,2005).Wolf-Rayet (WR)stars in particular,albeit rare and short-lived,make a significant contribution to their environment via the mechanical return of nuclear pro-cessed material to the interstellar medium (ISM)through their exceptionally powerful stellar winds.Metallicity,Z ,is a key factor in determining the number and subtype distribution of a WR population.Although the metallicity dependence of WR wind proper-ties still remains unclear,mass-loss prior to this phase has been established to depend on metallicity,with the lat-est models predicting ˙M∝Z ∼0.8for O stars (Vink et al.,2001).Evolutionary models for single stars predict that the minimum mass cut-offrequired for WR formation should decrease as metal content increases.It is antici-pated that the minimum mass required forprogression2L.J.Hadfield et al.:WR population of M83super-Solar metallicity,but its unfavourable inclination and large spatial extent makes surveying the complete WR population very challenging.In order to increase the vari-ety of galaxies sampled,our group has begun to look be-yond the Local Group(e.g.NGC300,Schild et al.,2003).Galaxies hosting substantive WR populations are known as‘WR galaxies’(Kunth&Sargent,1981; Schaerer et al.,1999b),where the number of WR stars ranges from∼35in NGC1569-A(Gonzalez Delgado et al., 1997)to2×104in Mrk309(Schaerer et al.,2000).Within specifically metal-rich environments,previous studies of WR populations have generally been restricted to in-tegrated spectra from bright star forming knots(e.g. Schaerer et al.,1999a)or H ii regions(e.g.Pindao et al., 2002).Here we present the results of a deep imaging and spectroscopic survey of the disk WR population within the metal-rich galaxy M83,in which WR signatures have previously been identified by Rosa&Richter(1988)and Bresolin&Kennicutt(2002).M83(NGC5236)is a massive,grand-design southern spiral(SBc(s)II)with on-going star formation in its spiral arms plus an active nuclear starburst(Elmegreen et al., 1998;Harris et al.,2001).M83is the principal mem-ber of a small galaxy group(∼11members)within the Centaurus A complex(Karachentsev et al.,2002). Located at a distance of4.5±0.3Mpc(Thim et al.,2003), its favourable inclination and apparently high metal abun-dance of log(O/H)+12=9.2(Bresolin&Kennicutt,2002) makes M83an ideal candidate for studies of massive stel-lar populations at high metallicity.More recently,oxygen abundances in metal-rich galax-ies have been revised downward(Pilyugin et al.,2004; Bresolin et al.,2004),such that M83may have a metal abundance closer to log(O/H)+12=9.0(Bresolin,2004, m.),i.e.approximately twice the Solar oxy-gen abundance of log(O/H)+12=8.66recently derived by Asplund et al.(2004).We present the results of an imaging and spectro-scopic survey of the WR content of M83using the ESO Very Large Telescope(VLT).The present paper com-plements the initialfindings of this study reported in Crowther et al.(2004,hereafter Paper I).In Sect.2we briefly describe the observations and data reduction tech-niques employed.Section3discusses the method followed to obtain a global WR population of M83.Sect.4dis-cusses the properties of metal rich WR stars with those of Local Group galaxies and evolutionary models.Finally, conclusions are drawn in Sect.5.2.Observations and data reductionWe have observed M83with the ESO Very Large Telescope UT4(Yepun)and Focal Reduced/Low Dispersion Spectrograph#2(FORS2).The detector con-sists of a mosaic of two2048×1024MIT/LL CCDs which in conjunction with the standard collimator provides a field-of-view6.8′×6.8′and an image scale of0.126′′/pixel. Photometric observations of M83were made between May–June2002with follow-up spectroscopic data being acquired during April–June2003.2.1.ImagingM83subtends12.9′by11.5′on the sky,preventing it be-ing imaged by a single FORS2frame.In order to obtain complete coverage,the galaxy was divided into four over-lapping regions,covering the NE(Field A),NW(B),SE (C)and SW(D)as indicated in Fig.1.Occulting bars were positioned in Field C to prevent detector saturation by bright foreground stars.The central15′′appears sat-urated on all images obtained,and as a result the WR population of the nucleus can not be discussed further.FORS2was used on2June2002to obtain narrow-band images with central wavelengths4684˚A,4781˚A and band widths of66˚A and68˚A respectively.These were obtained consecutively for each Field in seeing condi-tions between0.6–0.8′′1with individual exposures of 1800s.Theλ4684filter is coincident with the strong WR emission features which incorporates the N iii(λ4640˚A), C iii(λ4650˚A)and He ii(λ4686˚A)emission lines,whereas the latter samples a wavelength region relatively free from emission,providing a measure of the continuum level.In addition to these,2exposures(60s and600s) were taken using narrow-band on-and off-Hαfilters (λ6563,6665˚A,FWHM=61,65˚A)on16May2002. Finally,in order to supplement the primary dataset,2ex-posures(60s and120s)were also acquired using a Bessell Bfilter on21May2002.2.2.PhotometryImages were prepared following standard procedures i.e. debiased,flatfield corrected and cosmic ray cleaned. Photometry of individual sources within M83was per-formed using the package daophot,a point-spread func-tion(PSF)fitting routine within iraf.Absolute pho-tometry in the broad-band Bfilter was achieved with the aid of photometric standardfields Ru152and PG 1528+062(containing a total of10photometric standards, 11.9≤B≤16.3).For the narrow-band images such stan-dards are not available and photometric zero-points have been obtained by observing spectrophotometric standards LTT7987(B=12.2)and G138-31(B=16.5).The majority of our sources appear point-like on the ground-based images.However,a number of bright sources are surrounded by a faint,extended halo,which was not accounted for in the PSF photometry and as a result only a lower limit to the magnitude is given,based on PSF photometry.A further subset of the bright sources are spatially extended,indicating that PSF photometry is in-appropriate,as indicated in Table A1in the appendix.Typical formal photometric errors range between 0.02mag(∼18mag),0.05mag(∼20mag)and0.08magL.J.Hadfield et al.:WR population of M833Fig.1.Four combined HαVLT FORS2frames of M83. The overall size of the image is∼12′×12′.Thefields A, B,C and D used to image M83are marked.North is up and east is to the left of the image.(∼22mag).Significantly higher errors,of up to0.15mag, are obtained for regions of the galaxy where the back-ground levels are high,or they are located in spatially crowded regions.As a consistency check we have compared results ob-tained for the two Bessell B exposures(for which the PSF model was based on different template stars)and also de-rived magnitudes for objects which appear in multiple fields.Excellent agreement was observed in both cases, with results agreeing to within the formal errors.In a mi-nority of cases this was not achieved due to severe crowd-ing.2.3.Candidate SelectionWR candidates were identified by searching for He ii/ C iii excess emission(atλ4684)relative to the contin-uum(λ4781),i.e.a negative value of∆m=mλ4684-mλ4781.The optimal method of identifying suitable candi-dates was found to be via‘blinking’individualλ4781and λ4684frames together with the difference image obtained by subtracting theλ4781image from theλ4684frame.In total,283candidateλ4684emission sources were identi-fied.For75%of our candidates we have obtained a magni-tude in at least theλ4684filter.For cases where we did not obtain photometry,the object was either too faint or was located in a spatially crowded region.In addition,for a significant fraction of the fainter sources it was not pos-sible to measure aλ4871parison between mλ4781magnitude and mλ4684excess of the WR candidates located in M83. Regions which have been spectroscopically observed and subsequently eliminated or classified as WR regions are presented in the key.Regions which still await spectro-scopic observations are also marked.Sources for which PSF photometry was unavailable are not marked.Candidates were grouped according to continuum brightness,∆m,and association with underlying H ii re-gions.To ensure we spectroscopically observed a repre-sentative sample,a selection from each group was cho-sen for spectroscopic follow up.In Fig.2we show∆m as a function of continuum magnitude for the sources in which WR signatures were either spectroscopically con-firmed,rejected or no spectroscopy was obtained,i.e.the remaining candidates.The majority of confirmed sources have aλ4684excess between–1.5≤∆m≤–0.4mag,al-though a few do exhibit rather smaller values of∆m.In contrast,all rejected regions have∆m≥–0.2mag,sug-gesting that remaining candidates which display a moder-ateλ4684excess should represent regions that genuinely host WR stars,together with a subset of those for with ∆m∼0.0mag.2.4.SpectroscopySpectroscopic data was obtained using FORS2with the Multi Object Spectroscopy(MOS)mode.MOS datasets of individual WR candidates were obtained during seeing conditions of∼0.5–1.0′′,using a slit width of0.8′′.The CCD was binned by a factor of2in the dispersion di-rection,resulting in a dispersion of3.3˚A pixel−1with the 300V grism and a spectral resolution of∼7˚A,as mea-sured from comparison arc lines.The wavelength range of individual targets depended on their position within the MOS mask but typical wavelength coverage was∼3700˚A to∼7500˚A.MOS allows the spectra of up to19candidates to be recorded simultaneously.However,due to positional limi-tations this was generally restricted to∼15,supplemented where possible by H ii regions.In total,198candidates have been spectroscopically observed using17different MOS masks.To maximise continuum S/N,sources were4L.J.Hadfield et al.:WR population of M83 Table1.FORS2Multi Object Spectroscopy(MOS)ob-serving log for M83.2003-04-06D23×9000.62003-04-13D11×24000.7D33×5000.7D53×6000.52003-05-21C23×9000.9C33×2400.92003-05-26A2,B23×9000.7,0.8A53×6000.8A43×2400.6L.J.Hadfield et al.:WR population of M 8353.1.Interstellar reddeningEstimates of the interstellar reddening for our confirmed WR sources have generally been derived using measure-ments of the nebular H α(accounting for nearby [N ii ]emission)and H βfeatures present in the extracted spec-tra.Assuming Case B recombination theory for typi-calelectron densities of 102cm −3and a temperature of 104K (Hummer &Storey,1987),we obtain 0.2≤E(B-V)=c (H β)/1.46≤0.8mag for the majority of the sources,with a few outliers,and typical formal uncertainty of ±0.02mag.Where Balmer emission was observed,typi-cal H βequivalent widths lay in the range and ∼20to ∼150˚A .Consequently,the underlying stellar absorption components (≤1˚A at H β)are neglected.In 41sources no nebular lines were observed.For those with a well defined continuum,E(B–V)was estimated by assuming an intrinsic optical flux distribution equiv-alent to a late O-type star,with typical uncertainty of ±0.05–0.1mag.In 15cases,the continuum S/N was in-sufficient for this comparison and an average reddening of E(B–V)=0.5±0.3was adopted.Correction for redden-ing adopt a standard Seaton (1979)extinction law with R=3.1=A V /E(B-V).3.2.Spectral classificationIn order to classify and quantify the WR population within each region,we have fit Gaussian line profiles to the blue and yellow WR features,revealing line fluxes,equivalent widths and FWHM.An example of the fits to the blue and yellow WR features is presented in Fig.3,where a source (#74)hosting a mixed WN and WC pop-ulation is presented.In general,it was straightforward to distinguish be-tween WN (strong He ii λ4686)and WC subtypes (strong C iii λ4650and C iii λ5696and/or C iv λ5801-12).The following classification scheme was applied for further sub-division.In a minority of cases it was not possible to sep-arate the λ4650–λ4686features into individual compo-nents,and as a result an overall blend was measured.Since WC subtypes were assigned on the basis of λ5696and λ5812features,this did not prevent accurate classifica-tion.Late and early WN subtypes were assigned if He ii λ4686emission was accompanied by N iii λ4634−41or N v λ4603−20emission,respectively.If nitrogen lines were undetected,we assigned a WNE subtype if FWHM (He ii λ4686)>20˚A ,and WNL otherwise.For WC stars,WC4–6was assigned if C iv λ5801−12was present along with either weak or absent C iii λ5696.For 0.25≤F λ(C iii λ5696/C iv λ5801-12)≤0.8sources were classified WC7,and WC8–9if C iii λ5696was present,with C iv λ5801−12weak or absent.To ensure consistency with previous studies (e.g.Schaerer et al.,1999a;Bresolin &Kennicutt,2002;Chandar et al.,2004)we have derived WR popula-Fig.4.De-reddened spectral comparison between WC members in M 83with Galactic WC stars scaled to the distance of M 83(Galactic distances from van der Hucht (2001)).To avoid confusion,WCE and WC7sources are offset by 2×10−17erg s −1cm −2˚A −1whereas WC8and WC9spectra are offset by 1×10−17erg s −1cm −2˚A −1.6L.J.Hadfield et al.:WR population of M83Fig.5.De-reddened spectral comparison between WN complexes in M83with individual Milky Way WN stars scaled to the distance of M83(Galactic dis-tances from van der Hucht(2001)).To avoid confusion, individual sources are successively offset by1×10−17 erg s−1cm−2˚A−1.tions based on individual linefluxes adapted from Schaerer&Vacca(1998).As discussed in Paper I,we adopt He iiλ4686linesfluxes of5.2×1035erg s−1and 1.6×1036erg s−1for WN2-5and WN6-10stars,respec-tively.For WC stars,we adopt C ivλ5801linefluxes of 1.6×1036erg s−1and 1.4×1036erg s−1for WC4–6 and WC7stars,respectively,and a C iiiλ5696lineflux of7.1×1035erg s−1for WC8–9stars.WR contents of individual sources then follow,with populations rounded to the nearest integer(≥1).In one source(#117),we were unable to reliably extract the spectrum since it was located at the very edge of the slit,and so a measure of the reddening/lineflux was not possible.Nevertheless, broad He iiλ4686is clearly present,with no WC signa-ture,such that we indicate a population of≥1early-type WN star.In Fig.4we compare sources containing representa-tive late,mid and early WC stars from M83with ex-tinction corrected Milky Way counterparts,scaled to the distance of rge line widths amongst M83mem-bers hosting late WC stars are apparent,particularly for #32versus HD192103(WC8)and#81versus HD164270 (WC9).In contrast,sources containing WC4–7stars in-dicate similar line widths to individual Galactic parisons between sources containing WNL and WNE stars in M83and two Galactic counterparts are shown in Fig.5,revealing similar spectral morphologies. Other examples of sources hosting WN and WC popula-tions are presented in Fig.1of Paper I.3.3.The M83WR population–individual stars,binaries,complexes or clusters?What is the nature of the132sources in M83that are known to host WR stars?In Fig.6(a)we compare the spectroscopic continuum magnitude to the spectroscopic excess,∆m spec=mλ4684–mλ4781,for all sources.This is more complete than Fig.2,since it was generally possible to estimate the spectral mλ4781magnitude for the fainter sources,where PSF-photometry was not available.The brightest confirmed WR sources in our sample (mλ4781∼20mag)exhibit–0.3≤∆m spec≤0.0mag.Such values are consistent with luminous complexes,greatly di-luting the WR emission signature.In contrast,the faintest confirmed sources(mλ4781∼25mag)possess large spec-troscopic excesses of–2≤∆m spec≤–0.5mag,consistent with isolated,single or binary WR systems.Intermediate brightness sources span the full range in excess,corre-sponding to less luminous regions hosting a few WR stars to those containing large WR populations.Fig.6(b)compares the spectroscopicλ4686excess to the C iiiλ4650/He iiλ4686equivalent width,confirming the expected tight correlation between line strength and ∆m spec,where the scatter indicates the observational ac-curacy.Typical excesses of–0.2mag equate to small line equivalent widths of∼10˚A,whilst an excess of–1.0mag corresponds to∼100˚A,and the largest excesses equate to ∼500˚A.For comparison,single Galactic and LMC WR stars possess C iiiλ4650/He iiλ4686equivalent widths of 10–500˚A(WN subtypes)or150–2000˚A(WC subtypes).3.4.The global disk WR population of M83We identify1035±300WR stars,comprising564±170WC and471±130WN stars,within our132spectroscopically observed regions,where errors quoted here were obtained from simply adding individual uncertainties for all regions.The most important discovery of our spectroscopic sur-vey is the dominant late-type WC population of M83. Over half of the spectroscopically identified WR stars in M83fall into the WC8–9subtype,with few WC4–7 stars identified.For comparison,no WC8–9stars are ob-served in the SMC,LMC or M33and the total number of such stars in the Milky Way and M31is less than50 (van der Hucht,2001;Moffat&Shara,1987).The distri-bution among late-and early-type WN stars is more even, with WNL/WNE∼1.This value is much greater than that observed in the SMC(∼0)and LMC(∼0.25),but comparable to that of∼1.3determined for the Milky Way(van der Hucht,2001).How robust is this derived WR population for M83? For each source,we have propagated uncertainties in theL.J.Hadfield et al.:WR population of M837 parison between(a)the spectroscopic mλ4781magnitude and(b)line equivalent width of the C iiiλ4650/He iiλ4686WR features to the spectroscopic mλ4684excess.Two WC objects,#96and124,are not marked,since their spectra start longward of He ii.The left panel confirms that regions without WR signatures are clustered around∆m spec∼0±0.2mag,whilst regions with confirmed WR signatures span a wide range,up to ∆m spec∼−2.0mag.distance,reddening,photometry and lineflux measure-ments.Together,these translate to a typical uncertaintyof∼20−30%,or somewhat higher for regions in which aninterstellar reddening or a slit loss correction factor havebeen adopted.One of the main limitations in estimating the con-tent of an unresolved WR population is the conversionfrom WR lineflux to WR content.Given the large lateWC population identified in M83,we have reconsideredthe lineflux of individual WC8–9stars determined bySchaerer&Vacca(1998).From unpublished data for5Galactic,and2M31WC8–9stars,each with well deriveddistances,wefind a meanλ5696flux of5.1×1035ergs−1and4.7×1035ergs−1respectively.This is∼30%lower thanSchaerer&Vacca,and suggests that,if anything,we maybe underestimating the true WC population of M83.We have also estimated the WC population using thealternative C iiiλ4650line.Based on individual WRλ4650linefluxes of3.4×1036,4.5×1036and1.0×1035ergs−1for individual WC4–6,WC7and WC8–9subtypes,respec-tively(Schaerer&Vacca,1998),populations of individualsources were found to agree to within a factor of2,rela-tive to the yellow features.The total WC population wascalculated to be594using C iiiλ4650,in excellent agree-ment with that of564obtained from C iiiλ5696and C ivλ5808.Turning to the candidates for which spectroscopy wasnot obtained,all regions in Fig.2with∆m≤–0.3magcorrespond to spectroscopically confirmed WR complexes.Therefore,we would expect that at least25out of the49candidates,for whichλ4684andλ4781photometryis available,also possess WR stars.Adopting the samefraction for regions where PSF photometry is not avail-able,we expect≥50of the remaining89candidate regionsto contain WR stars.Indeed,#159has already been ob-served by Bresolin&Kennicutt(2002).Designated M83-5in their study,WR emission is spectroscopically confirmedand a population of2WCL and6WNL stars(scaled toa distance of4.5Mpc)is inferred from its line luminos-ity.On average,our confirmed sources host∼5WR stars,such that we expect∼250WR stars await identificationin M83,bringing the total disk population to∼1300.The inferred WR population of M83is greaterthan that known in the entire Local Group,to date(Massey&Johnson,1998).As anticipated from Figs.2and6,some sources host a single WR star,whilst oth-ers contain larger WR populations(∼10).Regions whichcontain an exceptionally large WR population will be dis-cussed in more detail in the next section.plexes hosting large WR populationsIn the Milky Way,the most massive open clusters(e.g.Arches,Westerlund1)host at most10–20WR stars(Blum et al.,2001;Negueruela&Clark,2005).Similarnumbers are observed in the largest H ii regions of M33,and30Doradus in the LMC.We identify10regions inM83with large(≥20),or mixed,WR populations.MixedWN and WC populations are observed in a total of5com-plexes,#66(8±2WNL,4±1WC7),#38(7±2WNL,21±6WCL),#41(14±4WNL,13±6WC7),#86(9±4WNL,24±10WCL)and#74which will be discussed sep-arately.Are the sources that host WR stars in M83com-pact clusters(e.g.Arches)or extended,giant H ii re-gions(30Doradus)?Massive compact clusters are gener-ally rare in normal disk galaxies,although M83is knownto host many examples,from HST imaging(Larsen,2004).Of the60bright H ii regions in M83identifiedby de Vaucouleurs et al.,between28–38host WR pop-ulations.Indeed,the3complexes hosting the largest WRpopulations are all associated with H ii regions identifiedby de Vaucouleurs et al..Optical spectroscopy of these8L.J.Hadfield et al.:WR population of M83were presented in Paper I,together with an estimate of their O starpopulation.Fig.7.5′′×5′′images of the WR cluster M83#74from VLT/FORS2and HST/ACS.Top Panel:λ4684filter,mid-dle panel:continuum subtracted Hαfilter,lower panel: F475Wfilter(WFC).North is up and east is to the left. It is apparent that the brightest Hαsource lies∼2′′to the S-W from the continuum(and WR)source.3.5.1.Source#74From our sample#74is exceptional,with230±50late-type WN and WC stars inferred from the de-reddened line fluxes(recall Fig.3).This source has the highest interstel-lar reddening of our entire sample with E(B-V)=1.0±0.03, although it is closest to the nucleus.However,the Hα/Hβnebular value is supported fromfitting its stellar contin-uum to a young(∼4Myr)instantaneous burst model at Z=0.04from Starburst99(Leitherer et al.,1999).In Paper I,we estimated a Lyman continuumflux of8×1051 s−1from the de-reddened Hαflux,such that#74has an ionizingflux equivalent to the giant H ii region30 Doradus.However,it possesses a WR content which is a factor of ten times larger,i.e.N(WR)/N(O)∼0.25versus 0.02in30Doradus.We have inspected archival HST/Advanced Camera for Surveys(ACS)Wide Field Camera(WFC)F475W datasets of M83(Proposal9299,P.I.H.Ford).This re-vealed that#74is very compact,with a FWHM of∼0.2 arcsec or∼4.5pc(for a distance of4.5Mpc).For H ii re-gions with solar or super-solar metallicities,WR signa-tures are expected to be present in bursts of age3–6Myr. We have compared the absolute F475W magnitude of#74 with evolutionary synthesis models for an instantaneous burst of age3–5Myr(Leitherer et al.,1999),from which we estimate a mass of1.4–2×105M⊙.Therefore,its mass and size indicate that it is a young massive compact clus-ter,or Super Star Cluster(Whitmore,2003).In Fig.7we present5×5arcsec(∼110×110pc) images of#74obtained with FORS2and ACS.It is ap-parent that the peak Hαsource,i.e.H ii region#35from de Vaucouleurs et al.(1983),lies∼2arcsec to the S-W of the brightest continuum source(the WR cluster).The spectrum presented in Fig.2of Paper I is that of the WR cluster,whilst the Hαflux,and corresponding O7V star content of∼810represents the integrated total from both regions.The WR cluster provides approximately1/3of the total H ii luminosity,such that the WR/O ratio of this region approaches unity,comparable to the WR clus-ter NGC3125-1(Chandar et al.,2004).3.5.2.Other Clusters in M83Larsen(2004)has identified∼80young massive clusters in M83based on HST/WFPC2images.Three such regions are in common with our catalogue of sources containing WR stars,namely n5236-607(#61),-617(#73)and-277 (#79),although none host more than a few WR stars. Larsen(m.)has compared the UBVI colours of these clusters with Solar metallicity Bruzual&Charlot (1993)models,suggesting age estimates of log(τ)= 6.20±0.51,6.90±0.54and9.89±1.87,respectively.The first two are fully consistent with a young cluster which contains WR stars,while the third suggests a dominant old population.Five additional clusters from Larsen(2004)are also in common with our remaining candidates,namely n5236-L.J.Hadfield et al.:WR population of M 839169(#193),-805(#179),-818(#163),-1011(#157)and -1027(#173).Of course,such candidates have the poten-tial to also host a large WR population -indeed three of these clusters appear young (∼1.5–6Myr)from UBVI pho-tometry (Larsen,m.),i.e.#193,#179and #157.Note that #179is one of two clustersfor which dynam-ical mass estimates has been made by Larsen &Richtler (2004).Follow-up spectroscopic observations would be re-quired for the identification of additional WR rich clusters.parisons with previous studiesTo date,there have only been two previous studies re-lating to WR stars within M 83.Rosa &Richter (1988)and Bresolin &Kennicutt (2002)have both studied stel-lar populations within M 83and identify six H ii re-gions which exhibit WR characteristics.Four of these have been re-examined in this study.Rosa &Richter ob-tain optical spectra with very poor signal-to-noise pre-venting a quantitative discussion,consequently we shall restrict any comparisons solely to results obtained by Bresolin &Kennicutt (2002).Both studies followed a similar methodology in estimating the WR population,except that Bresolin &Kennicutt adopted a distance of 3.2Mpc to M 83(versus 4.5Mpc adopted here).This introduces a factor of 2between intrinsic line luminosities observed in this study and that by Bresolin &Kennicutt.–#40(M83-2)–The derived WR population for this region is estimated to be 6±2WC8–9,contrasting that of 1–2WNL obtained by Bresolin &Kennicutt.We achieve a 3σdetection for the λ5696and λ5812car-bon features,suggesting that poor signal-to-noise in the original investigation prevented positive WC iden-tification.–#41(M83-3)–We confirm the detection of 14±4WNL stars identified in region M83-3.In addi-tion we estimate the presence of 13±6WC7stars.Bresolin &Kennicutt state that C iii may be present,but not at a significant level (versus 5σhere).–#74(M83-8)–Bresolin &Kennicutt failed to detect any WR emission in this H ii region.However,we find the largest individual WR population of any source,namely 230stars.As stated in Sect.3.5the WR emis-sion is offset by several arc-secs to the N-E of the peak H αemission.Since the Bresolin &Kennicutt concen-trated on bright H ii regions,their slit was probably centred on the peak H αemission,such that the WR signature was missed.–#103(M83-9)–Both investigations infer a late WN population.The present study obtains a population of 29±9WNL stars,in agreement with that estimated by Bresolin &Kennicutt after allowing for differences in the assumed distance.Fig.8.Distribution among WCL subtypes as determined using W λ(C iv 5808)/W λ(C iii 5696)versus FWHM (C iii λ5696)in ˚A .For comparison,Galactic (unpub-lished WHT,AAT and 2.3m ANU data),M31(unpub-lished WHT /ISIS data),M33(Abbott et al.,2004)and IC10(Crowther et al.,2003)WCL stars have been in-cluded.The subtype divisions marked are those derived by Crowther et al.(1998).Fig.9.Equivalent width (W λin ˚A )vs.FWHM (˚A )of the C iii λ5696for WCL stars in M 83and Local Group galaxies (identical dataset as presented in Fig.8).4.DiscussionWe have identified up to ∼200regions in the disk of M 83that host WR stars.We now compare the properties of WR stars at the high metallicity of M 83with those of Local Group galaxies,attempt to explain the dominant late subtypes amongst WC stars,and make comparisons with current evolutionary models.4.1.Properties of WR stars at high metallicityHow do the line strengths and widths of sources con-taining WR stars in M 83compare with those of other galaxies?In Fig.8,we show the classification ratio W λ(C iv 5808)/W λ(C iii 5696)versus FWHM (C iii 5696).Data for WC7–9stars in four Local Group galaxies are included,along with subtype boundaries as derived by。
冲击波聚心的英文
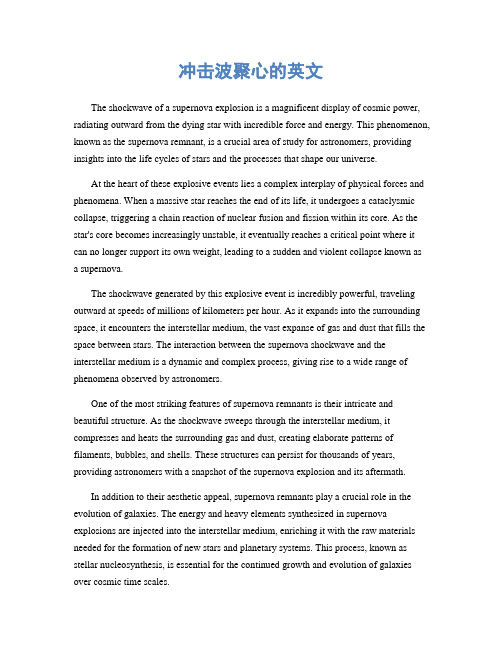
冲击波聚心的英文The shockwave of a supernova explosion is a magnificent display of cosmic power, radiating outward from the dying star with incredible force and energy. This phenomenon, known as the supernova remnant, is a crucial area of study for astronomers, providing insights into the life cycles of stars and the processes that shape our universe.At the heart of these explosive events lies a complex interplay of physical forces and phenomena. When a massive star reaches the end of its life, it undergoes a cataclysmic collapse, triggering a chain reaction of nuclear fusion and fission within its core. As the star's core becomes increasingly unstable, it eventually reaches a critical point where it can no longer support its own weight, leading to a sudden and violent collapse known as a supernova.The shockwave generated by this explosive event is incredibly powerful, traveling outward at speeds of millions of kilometers per hour. As it expands into the surrounding space, it encounters the interstellar medium, the vast expanse of gas and dust that fills the space between stars. The interaction between the supernova shockwave and the interstellar medium is a dynamic and complex process, giving rise to a wide range of phenomena observed by astronomers.One of the most striking features of supernova remnants is their intricate and beautiful structure. As the shockwave sweeps through the interstellar medium, it compresses and heats the surrounding gas and dust, creating elaborate patterns of filaments, bubbles, and shells. These structures can persist for thousands of years, providing astronomers with a snapshot of the supernova explosion and its aftermath.In addition to their aesthetic appeal, supernova remnants play a crucial role in the evolution of galaxies. The energy and heavy elements synthesized in supernova explosions are injected into the interstellar medium, enriching it with the raw materials needed for the formation of new stars and planetary systems. This process, known as stellar nucleosynthesis, is essential for the continued growth and evolution of galaxies over cosmic time scales.Furthermore, supernova remnants are also sites of intense physical processes, including the acceleration of charged particles to relativistic speeds. These high-energy particles can interact with magnetic fields and emit radiation across the entire electromagnetic spectrum, from radio waves to gamma rays. Studying the emission from supernova remnants allows astronomers to probe the underlying physics of particle acceleration and magnetic field dynamics in extreme environments.In recent years, advances in observational techniques have led to a deeper understanding of supernova remnants and their role in the universe. High-resolution imaging and spectroscopic observations have revealed the intricate details of shockwave dynamics and the distribution of heavy elements within these cosmic relics. Theoretical models and numerical simulations have provided valuable insights into the physical processes driving the evolution of supernova remnants and their impact on galactic ecosystems.Looking ahead, future observations with next-generation telescopes and space-based observatories promise to unveil even more secrets of supernova remnants. By studying these cosmic artifacts in greater detail, astronomers hope to unravel the mysteries of stellar death and the profound influence it has on the evolution of the cosmos. From the heart of a dying star to the farthest reaches of the universe, the shockwaves of supernova explosions continue to captivate our imagination and inspire scientific discovery.。
HUBBL E SPACE T EL ESCOPE NICMOS SPECTROSCOPY OF CHARONS LEADING AND TRAILING HEMISPHERES
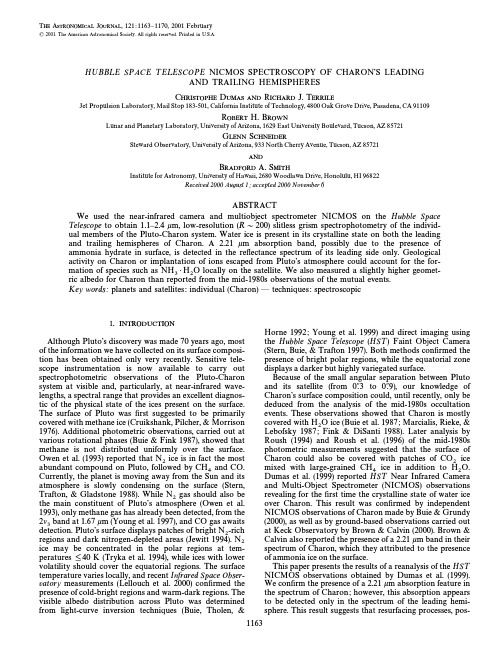
T HE A STRONOMICAL J OURNAL ,121:1163È1170,2001February(2001.The American Astronomical Society.All rights reserved.Printed in U.S.A.HUBBL E SPACE T EL ESCOPE NICMOS SPECTROSCOPY OF CHARONÏS LEADINGAND TRAILING HEMISPHERESC HRISTOPHED UMAS AND R ICHARD J.T ERRILEJet Propulsion Laboratory,Mail Stop 183-501,California Institute of Technology,4800Oak Grove Drive,Pasadena,CA 91109R OBERT H.B ROWNLunar and Planetary Laboratory,University of Arizona,1629East University Boulevard,Tucson,AZ 85721G LENN S CHNEIDERSteward Observatory,University of Arizona,933North Cherry Avenue,Tucson,AZ 85721ANDB RADFORD A.S MITHInstitute for Astronomy,University of Hawaii,2680Woodlawn Drive,Honolulu,HI 96822Received 2000August 1;accepted 2000November 6ABSTRACTWe used the near-infrared camera and multiobject spectrometer NICMOS on the Hubble Space Telescope to obtain 1.1È2.4k m,low-resolution (R D 200)slitless grism spectrophotometry of the individ-ual members of the Pluto-Charon system.Water ice is present in its crystalline state on both the leading and trailing hemispheres of Charon.A 2.21k m absorption band,possibly due to the presence of ammonia hydrate in surface,is detected in the reÑectance spectrum of its leading side only.Geological activity on Charon or implantation of ions escaped from PlutoÏs atmosphere could account for the for-mation of species such as locally on the satellite.We also measured a slightly higher geomet-NH 3ÉH 2O ric albedo for Charon than reportedfrom the mid-1980s observations of the mutual events.Key words:planets and satellites:individual (Charon)Ètechniques:spectroscopic1.INTRODUCTIONAlthough PlutoÏs discovery was made 70years ago,most of the information we have collected on its surface composi-tion has been obtained only very recently.Sensitive tele-scope instrumentation is now available to carry out spectrophotometric observations of the Pluto-Charon system at visible and,particularly,at near-infrared wave-lengths,a spectral range that provides an excellent diagnos-tic of the physical state of the ices present on the surface.The surface of Pluto was Ðrst suggested to be primarily covered with methane ice (Cruikshank,Pilcher,&Morrison 1976).Additional photometric observations,carried out at various rotational phases (Buie &Fink 1987),showed that methane is not distributed uniformly over the surface.Owen et al.(1993)reported that ice is in fact the mostN 2abundant compound on Pluto,followed by and CO.CH 4Currently,the planet is moving away from the Sunand itsatmosphere is slowly condensing on the surface (Stern,Trafton,&Gladstone 1988).While gas should also beN 2the main constituent of PlutoÏs atmosphere (Owen et al.1993),only methane gas has already been detected,from the band at 1.67k m (Young et al.1997),and CO gas awaits 2l 3detection.PlutoÏs surface displays patches of bright N 2-richregions and dark nitrogen-depleted areas (Jewitt 1994).N2ice may be concentrated in the polar regions at tem-peratures ¹40K (Tryka et al.1994),while ices with lower volatility should cover the equatorial regions.The surface temperature varies locally,and recent Infrared Space Obser -vatory measurements (Lellouch et al.2000)conÐrmed the presence of cold-bright regions and warm-dark regions.The visible albedo distribution across Pluto was determined from light-curve inversion techniques (Buie,Tholen,&Horne 1992;Young et al.1999)and direct imaging using the Hubble Space Telescope (HST )Faint Object Camera (Stern,Buie,&Trafton 1997).Both methods conÐrmed the presence of bright polar regions,while the equatorial zone displays a darker but highly variegated surface.Because of the small angular separation between Pluto and its satellite (from to our knowledge of 0A .30A .9),CharonÏs surface composition could,until recently,only be deduced from the analysis of the mid-1980s occultation events.These observations showed that Charon is mostly covered with ice (Buie et al.1987;Marcialis,Rieke,&H 2O Lebofsky 1987;Fink &DiSanti 1988).Later analysis by Roush (1994)and Roush et al.(1996)of the mid-1980s photometric measurements suggested that the surface of Charon could also be covered with patches of iceCO 2mixed with large-grained ice in addition to CH 4H2O.Dumas et al.(1999)reported HST Near Infrared Camera and Multi-Object Spectrometer (NICMOS)observations revealing for the Ðrst time the crystalline state of water ice over Charon.This result was conÐrmed by independent NICMOS observations of Charon made by Buie &Grundy (2000),as well as by ground-based observations carried out at Keck Observatory by Brown &Calvin (2000).Brown &Calvin also reported the presence of a 2.21k m band in their spectrum of Charon,which they attributed to the presence of ammonia ice on the surface.This paper presents the results of a reanalysis of the HST NICMOS observations obtained by Dumas et al.(1999).We conÐrm the presence of a 2.21k m absorption feature in the spectrum of Charon;however,this absorption appears to be detected only in the spectrum of the leading hemi-sphere.This result suggests that resurfacing processes,pos-11631164DUMAS ET AL.Vol.121TABLE1G EOMETRY OF THE P LUTO-C HARON S YSTEM DURING THE1998J UNE““C AMERA3C AMPAIGNÏÏProperty Jun11,UT1301a Jun14,UT1456aHeliocentric distance(AU)................30.068430.0692Geocentric distance(AU).................29.104929.1190Sub-Earth latitude(deg)...................[21.4[21.3PlutoÏs sub-Earth longitude(deg)........99.6(max.light curve)273.2(min.light curve)CharonÏs sub-Earth longitude(deg)......279.7(trailing side)93.2(leading side)Separation(arcsec).........................0.9080.917CharonÏs position angle(deg)............169.95347.65Spectral separation(pixels)............... 2.55(\0A.517) 2.70(\0A.548)a Time at beginning of G206series.sibly involving Pluto,are currently occurring on Charon.A mechanism is discussed that involves bombardment of CharonÏs surface by ions escaping from PlutoÏs atmosphere.2.OBSERVATIONSWe report spectroscopic observations of the Pluto-Charon system obtained in1998on June11and14(HST program GTO7223)at CharonÏs maximum elongation using HST and its near-infrared camera and multiobject spectrometer NICMOS(Thompson et al.1998).The details of the geometry of the Pluto-Charon system are described in Table1.Planetocentric coordinates were calculated for both dates using the1994IAU model(Davies et al.1995). NICMOS has three256]256HgCdTe low-noise detec-tors covering a0.8È2.4k m spectral range with pixel scales ranging from43mas pixel~1(camera1)to203mas pixel~1 (camera3).Camera3(NIC3)is equipped with a set of three grisms,an optical setup combining a prism and a grating, providing low-resolution(R D200)slitless spectro-photometric capabilities.In this paper,we discuss the results obtained using NIC3and the G141and G206 grisms,spanning a spectral range from 1.1to 2.4k m. Though NIC3normally produces slightly afocal images, our data were obtained during the1998June““camera3 campaign,ÏÏduring which HSTÏs secondary mirror was adjusted to achieve optimal optical performance.Pluto and Charon were separated by for the dates of our obser-0A.9vations.However,because of HST scheduling constraints, the line joining the binary system could not be oriented perpendicular to the dispersion direction;hence,the spatial separation of the spectra was reduced to(Table1).0A.5Table2summarizes the exposure parameters for the G141 and G206grisms.Because the wavelength solution of each spectrum is a function of the position of the science target on the detector,we initially obtained an image of Pluto-Charon through the F150W broadÐlter.Two spectra of Pluto-Charon(320s per exposure)were then recorded with the G141grism.A small telescope o†set was implemented between each exposure to collect data from di†erent loca-tions on the detector and to reduce the contamination ofTABLE2G RISM O BSERVATION P ARAMETERSParameter G141G206Spectral coverage(k m)...... 1.1È1.9 1.4È2.5Number of exposures (212)Total exposure time(s)......639.9767.4NICMOS sequence..........STEP64STEP16our data fromÑat-Ðeld residual e†ects,as well as to sample around bad pixels.The last step was to acquire the long-wavelength spectra using the G206grism.Because the G206 background level was D150times higher than for the G141 grism,shorter exposures were required in order to avoid saturation.We recorded a series of12spectra(64s each)at four di†erent locations on the detector(three images per position of the telescope).3.DATA REDUCTIONThe extraction and absolute photometric calibration of the spectra of Pluto and Charon were achieved using the ““NICMOSlookÏÏsoftware(Pirzkal&Freudling1998; McCarthy et al.1999),developed at the Space Telescope European Coordinating Facility for reduction of the NICMOS spectroscopic data.3.1.Separation of Pluto and CharonTheÐrst step in data reduction was to subtract the back-ground from the G206images.The background was esti-mated from a median combination of the set of four-position dithered G206images.The low background level for the G141grism was simplyÐtted during the extrac-tion.Because the spectra of the two objects partially overlap,we estimated their individual contributions and created,for each original spectrum,two images containing separate synthetic spectra of Pluto and Charon.Figure1 shows the result ofÐtting the contribution of Pluto and Charon along one detector column.Because the pixel~10A.2scale of NIC3oversamples the point-spread function(PSF), each image wasÐrst rebinned to aÐner grid(rebin factor\8)via bicubic convolution interpolation(Park& Schowengerdt1983).The angle of dispersion of the spectra with respect to the detectorÏs horizontal axis was measured, as well as the location where the spectrum was illuminating the exact center of one pixel.From the knowledge of these two parameters,we could estimate the width of PlutoÏs and CharonÏs PSFs.The separated synthetic spectra of Pluto and Charon were obtained by adjusting the amplitude and position of the two PSFs in order to minimize the variance of the di†erence between the original and the synthetic pro-Ðles(Fig.1).This measurement was made over the range of pixels covered by the two objects,and a goodÐt was reached after only three iterations.The process returned two images corresponding to the separated spectra of Charon and Pluto,which were rebinned back to the orig-inal resolution.The maximum count rate for Pluto in a single G206exposure was near330e~s~1,while the residual count rate was in the^13e~s~1range(NIC3 gain\6.5e~ADU~1).The residual image(original imageNo.2,2001NEAR-IR SPECTROSCOPY OF CHARON 1165F IG .1.ÈCross section of a rebinned G206spectrum of Pluto-Charon along the spatial direction (y -axis of the detector).The original proÐle is noted with diamonds,while the synthetic proÐle of Pluto plus Charon is indicated by the red line.The residual of the Ðt (original proÐle minus synthetic)is represented with a black line.For each original image,two synthetic images are produced by Ðtting the individual contributions of Pluto and Charon along the detector columns (three iterations of the Ðtting algorithm).The synthetic images are then used to extract the indi-vidual spectra of Pluto and Charon.minus synthetic)was used to improve the models of PlutoÏs and CharonÏs spectra.The total Ñux in a detector pixelF T due to Pluto and Charon is simply (F P )(F C )F T \F P ]F C,and the residual value for one particular pixel is res \F origwhere is the Ñux of the original image for this[F T ,F orig pixel.Then the corrected Ñuxes for the Ðts of Pluto and Charon are respectively and F P @\F P ](F P /F T )res F C@\This correction,performed individually F C ](F C /F T )res.forall pixels,allows us to conserve the original total Ñux of Pluto and Charon while correcting the models for the uncertainties in the individual pixel response and the exact position of the PSF with respect to the center of the pixel.3.2.Extraction of the SpectraAt this stage,the data have been processed in such a way that we can extract and photometrically calibrate the G141and G206spectra of Pluto and Charon.Version 2.9.2of NICMOSlook (Freudling &Pirzkal 1998;Pirzkal &Freudling 1998)was used for this purpose.The wavelength solution for all NICMOS grisms as a function of the posi-tion of the object on the detector has been parameterized.The only adjustment we made consisted of accounting for the planetÏs apparent motion,which had the e†ect of modifying the original position measured from the F150W image.The NICMOS detectors do not have spatially Ñat responses across their pixels.Thus,the Ñux measured in an undersampled pixel depends upon the location of the PSF core with respect to the pixel center.It was therefore neces-sary to extract the spectra using a model of the intrapixel response function (IPRF;see Lauer 1999for a detailed description).The average e†ect of the IPRF was larger for ““camera 3campaign ÏÏobservations than for observations carried out when the PSF was not exactly in focus for NIC3.Similarly,because the PSF is better sampled at longer wavelengths,the correction for the G206grism data was smaller than for the G141grism.We used the obser-vations of the solar analog P330-E collected during the 1998June ““camera 3campaign ÏÏ(HST program 7959)to determine the amplitude of a characteristic IPRF correction to apply to our G206and G141spectra.The scatter of the data points was measured before and after applying the IPRF correction.For this purpose,we deÐned the mean spectrum for each grism and subtracted it from the individ-ual spectra to calculate the means of the standard devi-ations over a deÐned range of wavelengths.After applying the IPRF,we measured a decrease in the means of the standard deviations of 10%and 40%,respectively,for the G206and G141grisms.3.3.Calibration3.3.1.Spectral CalibrationGiven that the wavelength solution for each spectrum is a function of the target location on the detector,the estima-tion of this location (from the F150W image and the appar-ent motion of the object)provides an additional source of error for the spectral calibration.The wavelength sampling of the spectra after extraction is 10nm pixel ~1(G206grism).Therefore we Ðrst rebinned the individual spectra to a Ðner grid prior to co-registering them (this process was similarly applied to the spectrum of the solar analog P330-E).The spectra of P330-E and Pluto-Charon were then co-registered,using the absorption bands visible in both spectra as Ðducial.Because of the lower signal-to-noise ratio (S/N)of the G206data,and the deep bands of methane and water present at these wavelengths,the co-registration process returned a less accurate wavelength calibration for the G206grism than for G141.The accuracy of the wavelength calibration of the spectra is of the order of 20nm for the G206grism,slightly better at shorter wave-lengths (G141grism).This is illustrated by the small shifts (¹20nm)in wavelength that can be measured for the 1.85k m band of methane and the 2.05k m band of water in Figure 2.Smaller wavelength shifts can also be noticed for the G141grism.Such shifts result from the uncertainty in the wavelength calibration of our data set and do not rep-resent any real hemispheric variations in the physical state of the surface ices.3.3.2.Radiometric CalibrationFor each pixel,the response function in wavelength used to Ñat-Ðeld the spectra was determined by interpolating the narrowband Ñat Ðelds (*j /j D 1%)obtained as part of the standard NICMOS Cycle 7calibration program.The inverse sensitivity curves (used by NICMOSlook to convert the spectral Ñux into millijanskys)were obtained for each grism from observations of the calibrator stars P330-E and G191-B2B collected during the 1998June ““camera 3cam-paign.ÏÏThe intensities of the individual G206spectra were adjusted to minimize the scatter in our data set,and the 1p errors represent the residual dispersion after parison of the G141and G206spectra shows a mis-match in the overlap region,and we estimate the G206Ñux to be 12%higher than the G141Ñux after ing recent ground-based observations of Pluto carried out by Owen et al.(1993)and recalibrated in geometric albedo by Roush et al.(1996),we Ðnd that the G141grism calibration is best matched by the ground-based results,giving geometric albedos of p D 0.55and p D 0.7at 1.9k m for the minimum and maximum of PlutoÏs light curve,respectively.The precision of the Ñux calibration returned1166DUMAS ET AL.Vol.121by the G141grism is higher because its lower background makes the extraction of the photometric calibrators more accurate.We therefore selected the G141grism as the refer-ence for our photometric calibration.Our spectra of Pluto and Charon were then divided by the spectra of the solar analog P330-E (which were extracted using NICMOSlook as described above).After correction of our spectra for the solar spectrum,we calibrated the spectra of Pluto and Charon in geometric albedo using the solar Ñux given by Labs &Neckel (1968)and the radii for Pluto (1151^4km)and Charon (591^5km)determined by Reinsch,Burwitz,&Festou (1994).An independent reference for the solar Ñux (Thekekara 1973)was used and returned the same cali-bration values.4.RESULTS AND DISCUSSIONFigure 2shows the calibrated NICMOS spectra of Pluto and Charon obtained for the two dates 1998June 11and 14.The spectra have been slightly smoothed (convolution with a 2pixel wide Gaussian)in this Ðgure in order to make their comparison easier.The original spectra with error bars are presented below,along with the modeling results in °4.2.2.4.1.PlutoThe focus of this paper being Charon,we will not provide a detailed model and discussion for the case of Pluto (Fig.2a )and will refer to et al.(1999)for recent modeling Douteof PlutoÏs spectra.Nevertheless,we will note,as a proof of the validity of the extraction procedure described above,that (1)the two grism spectra are self-consistent in the 1.60È1.85k m overlap region and (2)there is excellent agreement between our NICMOS spectra and the higher resolutionspectra obtained by et al.(1999).The strong bands of Doutemethane ice are clearly visible,as well as the 2.15k m dip due to particularly for the spectrum that corresponds toN 2,minimumof PlutoÏs light parison of our spectra at both light-curve minimum and maximum with the higher resolution ground-based spectra obtained at UKIRT by Cruikshank et al.(1997)also shows an excellent agreement between the two data sets for the 1.9k m ““continuum ÏÏregion.At shorter wavelengths,we note that our determi-nation of PlutoÏs albedo is slightly higher than that reported by the UKIRT measurements,but comparison of the rela-tive 1.22k m/1.9k m ratio of the continuum between CH 4our calibrated NICMOS spectra and recent ground-based data obtained at Keck (Brown &Calvin 2000)conÐrms that the continuum level for the blue end of the spectra is higherF IG .2.ÈG141and G206spectra of (a )Pluto and (b )Charon obtained during the ““camera 3campaign ÏÏof 1998June.The species responsible for the absorption bands detected in our spectra are noted on the Ðgures.Near-infrared spectroscopy of Charon shows that the 1.65k m feature of crystalline water ice is visible for both the leading and trailing sides.The 2.21k m absorption reported from Keck observations of Charon by Brown &Calvin (2000)is visible in the HST NICMOS spectrum,but for the leading hemisphere only.Whatever compound is responsible for(NH 3?)this absorption,it is more abundant on the leading sideof the satellite than on its trailing side.than previously reported.Additional discussion about the Ñux calibration of our NICMOS data is presented in °4.2.2.Table 3presents the broadband photometric measurements derived from our spectroscopic data set for both obser-TABLE 3N EAR -I NFRARED P HOTOMETRY OF P LUTO -C HARON P LUTO (mag)C HARON (mag)D ATE (1998)J a H b K c J a H b K c Jun 11......12.69^0.0112.66^0.0113.09^0.0414.74^0.0314.70^0.0214.81^0.12Jun 14......12.83^0.0212.81^0.0213.19^0.0414.70^0.0414.63^0.0414.81^0.10N OTE .ÈThese measurements are derived from the spectra in Fig.3.The error bars in magnitude reÑect only the 1p uncertainty plotted in the Ðgure.The absolute photometric accuracy of the grisms in NIC3is itself evaluated to be in the 5%È10%range.a J :1.15È1.35k m;0mag \1576.2Jy for k m.j eff \1.25b H :1.50È1.80k m;0mag \1018.6Jy for k m.jeff \1.65c K :2.00È2.40k m;0mag \672.8Jy for k m.j eff\2.20No.2,2001NEAR-IR SPECTROSCOPY OF CHARON 1167vation dates.We measure a magnitude di†erence *KD 0.1between maximum and minimum of PlutoÏs light curve,which agrees with previous ground-based observations.4.2.CharonFigure 2b compares the calibrated spectra of the leading and trailing hemispheres of Charon and shows that (1)water ice appears to be in its crystalline state on both hemi-spheres,(2)the HST NICMOS geometric albedo is slightly higher than reported from analysis of the mid-1980s mutual occultation events,and (3)spectral features characteristic of species such as hydrogen cyanide (HCN)or,more probably,ammonia hydrate are present in the spectrum(NH 3ÉH 2O)of CharonÏs leading side.4.2.1.Crystalline Ice versus AmorphousThe spectra of Figure 2b show the 1.65k m spectral feature characteristic of crystalline water ice for both the trailing and leading hemispheres of the satellite.Water ice has also been found in its crystalline state on the surfaces of the large satellites of Uranus (Grundy et al.1999).This suggests that resurfacing processes can occur in the outer solar system at a faster rate than required for water ice to turn into its amorphous state under the action of solar irradiation (Strazzulla et al.1992).A possible mechanism,proposed by Brown &Calvin (2000),implicates vapor-ization of the outermost layers of water ice on Charon by micrometeorite bombardments and recondensation of this ice into its crystalline state over the surface of the satellite.Figure 2b also shows di†erences between the spectral response of CharonÏs leading and trailing hemispheres.In particular,the spectral slope at short wavelengths is stron-ger for the leading side,which could be directly linked to the nature of the neutral absorber in surface.Also,the depth of the 1.55k m water band is shallower for the leading side,which might indicate hemispheric variations in the grain size distribution of water ice,although we would expect a similar behavior for the 2.02k m water band.The 2.21k m feature in the spectrum of CharonÏs leading side will be discussed below in °4.2.3.4.2.2.Geometric Albedo of CharonTable 3presents the broadband photometric measure-ments derived from the spectra of CharonÏs trailing and leading sides.The K -band magnitude di†erence between the hemispheres is within the uncertainty of our measure-ments,conÐrming that the leading and trailing sides of Charon have a similar brightness.The calibration of our HST NICMOS spectra returns a value for CharonÏs geo-metric albedo of p D 0.42^0.05in the 1.8k m water contin-uum region (Fig.2b ),which is slightly higher than the p D 0.34^0.05measurement (Roush et al.1996)derived from the mid-1980s mutual events (although both error bars overlap).The di†erence in CharonÏs geometric albedo reported in this paper is small,but larger than the uncer-tainty of the NICMOS grism calibration.The latter is esti-mated to be a few percent higher than the 5%È10%range reported by Freudling &Pirzkal (1998),mainly because of the processes applied to our data to separate the contribu-tions of Pluto and Charon.Furthermore,the good agree-ment between the NICMOS determination of PlutoÏs geometric albedo (Fig.2a )and earlier ground-based mea-surements supports the absolute calibration of our data.This sensibly higher determination of CharonÏs albedocannot be produced by residual contamination from the planet.Indeed,the immediate consequence would be to underestimate PlutoÏs albedo,whereas our measurements agree with the ground-based results.The slightly higher NICMOS albedo is consistent (within the error bars)with the earlier results and might simply reÑect larger uncer-tainties than previously estimated in CharonÏs diameter and in the photometric calibration of the mutual-event data.Nevertheless,we cannot entirely rule out the possibility that the di†erences measured in the albedos of Pluto and Charon could result from systematic errors in the radio-metric calibration returned by the NICMOSlook package.If the increase in albedo is real,then it might suggest time-variable phenomena over Charon,such as seasonal changes occurring while the system is moving away from the Sun.Also,the satellite is now observed with an aspect angle 10¡larger than during the 1987mutual events,presenting a slightly larger apparent cross section of its southern polar region.The present geometry could contribute to an increase in albedo if the southern polar region is made of material that exhibits a high reÑectivity at these parison with other icy satellites of the outer solar system is possible.Near-infrared spectroscopy of the large satellites of Saturn returns geometric albedo measure-ments in the range p D 0.2(Hyperion)to p D 1.0(Mimas).The NICMOS observations show that CharonÏs albedo of p D 0.4at 1.8k m is very similar to the albedo of IapetusÏs trailing side,which is typical of a ““dirty ice ÏÏcomposition.Figure 3shows the result of modeling the reÑectance spectra of CharonÏs leading and trailing hemispheres with an intimate mixture (D 90%)of crystalline water ice (Grundy &Schmitt 1998)and D 10%of a spectrally blue component,the latter being required to match the contin-uum level at short wavelengths.In this model,the water ice is made of 30k m grains while the blue component corre-sponds to 10k m particles having a reÑectance spectrum decreasing linearly from 8%to 6%over the 1.0È2.7k m region.Both spectra of Charon are reasonably well matched by the spectrum of pure water ice,except for the 2.21k m band and the short-wavelength region (CharonÏs trailing side).The modeling results do not require the addi-tion of ice or ice to improve the Ðnal Ðt.CO 2CH 44.2.3.T he L eading Hemisphere of CharonThe HST NICMOS spectrum of CharonÏs leading side(Fig.3b )is identical to the spectrum of the same side of the satellite obtained at Keck by Brown &Calvin (2000).It displays the same 2.21k m spectral feature that was attrib-uted to the presence of ammonia hydrate on the surface of the satellite.This additional and independent detection of the 2.21k m band suggests that this spectral feature is real and rules out the possibility that it could be produced by residual contamination from Pluto.The Ðrst obvious candidate for this 2.21k m feature is ice itself.Although we do not expect methane ice to CH 4still be present over CharonÏs surface,we nevertheless modeled the spectrum of CharonÏs leading side (Fig.4)with an intimate mixture of water ice (D 78%;30k m grain size),methane ice (D 15%;30k m grain size),and D 7%of the spectrally blue compound described above.The model pre-sented in Figure 4shows that adding methane ice to the mixture does not provide a good match to the features observed in the spectrum of CharonÏs leading side.The small amounts of methane used in the model can reproduce。
Early Type Galaxies in the Mid Infrared a new flavor to their stellar populations
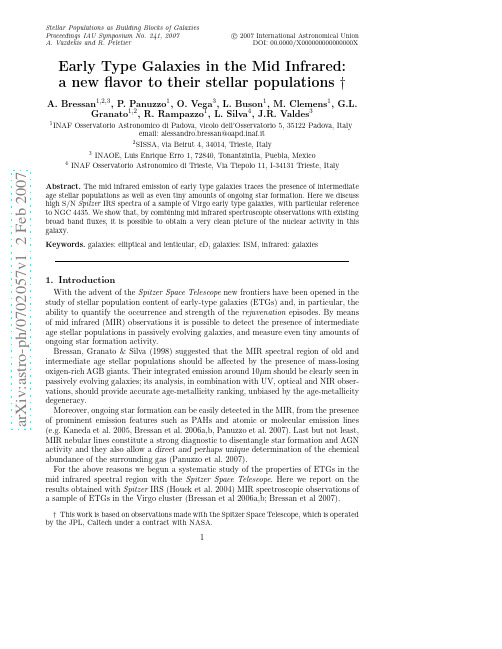
a rXiv:as tr o-ph/7257v12Fe b27Stellar Populations as Building Blocks of Galaxies Proceedings IAU Symposium No.241,2007A.Vazdekis and R.Peletier c 2007International Astronomical Union DOI:00.0000/X000000000000000X Early Type Galaxies in the Mid Infrared:a new flavor to their stellar populations †A.Bressan 1,2,3,P.Panuzzo 1,O.Vega 3,L.Buson 1,M.Clemens 1,G.L.Granato 1,2,R.Rampazzo 1,L.Silva 4,J.R.Valdes 31INAF Osservatorio Astronomico di Padova,vicolo dell’Osservatorio 5,35122Padova,Italy email:alessandro.bressan@oapd.inaf.it 2SISSA,via Beirut 4,34014,Trieste,Italy 3INAOE,Luis Enrique Erro 1,72840,Tonantzintla,Puebla,Mexico 4INAF Osservatorio Astronomico di Trieste,Via Tiepolo 11,I-34131Trieste,Italy Abstract.The mid infrared emission of early type galaxies traces the presence of intermediate age stellar populations as well as even tiny amounts of ongoing star formation.Here we discuss high S/N Spitzer IRS spectra of a sample of Virgo early type galaxies,with particular reference to NGC 4435.We show that,by combining mid infrared spectroscopic observations with existing broad band fluxes,it is possible to obtain a very clean picture of the nuclear activity in this galaxy.Keywords.galaxies:elliptical and lenticular,cD,galaxies:ISM,infrared:galaxies2Bressan,Panuzzo,Vega et al.parison between the observed SED of the central region of NGC4435and the GRASIL model.The thick solid line represents the model for the total SED,i.e.the starburst component plus the old stellar component;the three dots-dashed dark green line represents the contribution from the old stellar population,and the dashed blue line represents the total contribution from the burst of star formation,the dotted red line represents the emission from molecular clouds,the dot-dashed green line represents the diffuse medium emission and the thin solid cyan line denotes the emission from stars of the starburst component without applying the extinction from dust.Thefilled red circles are the broad band data.Left:Comparison from 0.1µm to100MHz.Right:Comparison for the MIR wavelengths.The thickest solid blue line represents the IRS Spitzer spectrum.2.Early-type galaxies in the mid infrared.Eighteen ETGs among those that define the colour-magnitude relation of the Virgo cluster(Bower,Lucy&Ellis1992)were observed in standard staring mode with the low resolution IRS modules between5and20µm,in January and July2005.The calibration and spectra extraction procedures are discussed in detail in Bressan et al.(2006a).The spectra of these galaxies are shown in Bressan et al.(2006a)and Bressan et al.(2007). For thirteen galaxies(76%)of our sample,the MIR spectrum is characterized by the presence of a broad emission features above10µm,without any other narrow emission feature.The analysis of the IRS spectra indicates that the10µm feature has an extended spatial distribution;moreover its spatial distribution is consistent with that obtained below8µm,where the spectra are dominated by stellar photospheres.This result has been confirmed by the analysis of Spitzer IRS Peak-Up imaging observations in the blue (16µm)filter of selected galaxies(Annibali et al.in preparation).It is also in agreement with previous ISOCAM observations that indicated spatially resolved emission at both 6.7and15µm(Athey et al.2002,Ferrari et al2002,Xilouris et al.2004).In view of these considerations and based on preliminaryfits with our models of passively evolving old simple stellar populations,we argued that we have detected the10µm features,due to silicate emission from the circumstellar envelopes of mass losing AGB stars,as predicted by Bressan et al.(1998).Bressan et al.(2007)have recently shown that the10µm feature observed in early type galaxies is similar in shape but about a factor four larger than the semi empirical one obtained for the globular cluster47Tuc,consistent with a metallicity variation of the same order.We are now computing new isochrones and SSP models that account for a more realistic description of the AGB phase and of their dusty envelopes. Among bright Virgo cluster ETGs observed by our team,four galaxies(24%)show various levels of activity.NGC4636(optically classified as a LINER)shows low ion-ization emission lines([ArII]7µm,[NeII]12.8µm,[NeIII]15.5µm and[SIII]18.7µm)on a continuum similar to other passive galaxies.NGC4486(M87)shows the same emission lines on a continuum dominated by the AGN emission above8µm.The broad contin-Early Type Galaxies in the Mid Infrared3 uum feature above10µm in M87could be caused by silicate emission from the dusty torus(Siebenmorgen et al.2005,Hao et al.2005).NGC4550shows some PAH emission features while the MIR SED of NGC4435is characteristic of a star forming object.3.The panchromatic SED of NGC4435NGC4435is an S0galaxy interacting with NGC4438and it hosts a circumnuclear disk.Panuzzo et al.(2007)combined the Spitzer IRS spectra of NGC4435with IRAC and MIPS archival data and existing broad band measurements from X-ray to radio wavelengths to obtain an accurate panchromatic spectral energy distribution(SED)of this galaxy.The SED was analysed with GRASIL(Silva et al.1998)and well reproduced at all wavelengths.The analysis shows that the circumnuclear disk experienced a burst of star formation activity which is now fading.The IRS data themselves provide precise answers on important questions such as the nature of the nuclear activity suspected from optical(Ho et al.1997)and X-ray (Machacek et al.2004)observations,and the metallicity of the gas in the circumnuclear disk.We fail to detect any high excitation nebular emission lines in the IRS spectrum;the [NeIII]15.5/[NeII]12.8ratio constrains the contribution of a possible AGN to the ionizing flux to be less than2%.The upper limit on the temperature derived from H2S(1)and S(2)rotational lines is lower than expected for AGN excitation and PAH features are well reproduced by star formation models.Moreover,the X-ray emission is within the range expected from X-ray binaries in an advanced phase of the starburst.As for the metallicity of the nuclear disk,the comparison of observed MIR nebular lines with those predicted by the GRASIL model(Panuzzo et al2003)indicates that it is almost solar.This is one of thefirst accurate direct estimates of the gas metallicity in ETGs.The age of the starburst,∼180Myr,corresponds to the epoch of the onset of the interaction with NGC4438derived from dynamical simulations(Combes et al.1988). The mass of stars born during the starburst(∼1.22×108M⊙)amounts to about1.5% of the stellar mass sampled by the central5arcsec aperture.4.ConclusionsWe have obtained with Spitzer IRS mid infrared spectra of ETGs selected along the colour-magnitude relation of the Virgo cluster.The mid infrared SED of most of our ETGs shows a clear broad emission around10µm and longward as predicted in Bressan et al.(1998)which is likely due to dusty mass losing AGB stars.In the remaining fraction of galaxies(24%)we detect signatures of activity at various levels.The analysis of the IRS spectrum of NGC4435testifies to the superb capability of Spitzer to probe the nature of this type of activity and supports the notion that ETGs with relatively strong hydrogen absorption features are due to recent small rejuvenation episodes,rather than being the result of delayed galaxy formation(Bressan et al.1996).AcknowledgementsA.B.,G.L.G.and L.S.thank INAOE for warm hospitality.ReferencesAthey,A.,Bregman,J.,Bregman,J.,Temi,P.,&Sauvage,M.2002,ApJ,571,2724Bressan,Panuzzo,Vega et al.Bower,R.G.,Lucey,J.R.,Ellis,R.S.1992,MNRAS,254,601Bressan,A.,et al.2006,ArXiv Astrophysics e-prints,arXiv:astro-ph/0701620Bressan,A.,et al.2006,ApJl,639,L55Bressan,A.,et al.2006,ArXiv Astrophysics e-prints,arXiv:astro-ph/0604068Bressan,A.,Granato,G.L.,Silva,L.1998,AA,332,135Bressan,A.,Chiosi,C.,&Tantalo,R.1996,A&A,311,425Combes,F.,Dupraz,C.,Casoli,F.,&Pagani,L.1988,A&A,203,9Ferrari,F.,Pastoriza,M.G.,Macchetto,F.D.et al.2002,A&A,389,355Hao,L.,et al.2005,ApJl,625,L75Ho,L.C.,Filippenko,A.V.,&Sargent,W.L.1997,ApJS,112,315Houck,J.R.2004,ApJS,154,18Kaneda,H.,Onaka,T.,&Sakon,I.2005,ApJl,632,L83Machacek,M.E.,Jones,C.,&Forman,W.R.2004,ApJ,610,183Panuzzo,P.,et al.2007,ApJ in press(astro-ph/0610316)Panuzzo,P.,Bressan,A.,Granato,G.L.,Silva,L.,&Danese,L.2003,A&A,409,99 Siebenmorgen,R.,Haas,M.,Kr¨u gel,E.,&Schulz,B.2005,A&A,436,L5Silva,L.,Granato,G.L.,Bressan,A.,&Danese,L.1998,ApJ,509,103Xilouris,E.M.et al.2004,A&A,416,41DiscussionMeixner:I’m happy to see that someone is including dust in their models.You say that the10µm emission in the elliptical is extended.Is your interpretation that the AGB population is extended?Is the extended emission consistent with the AGB population as traced by near-IR photometry?Bressan:Our IRS data analysis pipeline allows an accurate check of the spatial dis-tribution of the emission.In the passive galaxies,the emission looks extended at all wavelengths.The spatial extension is consistent with IRAC and2MASS images.The preliminary analysis of our Spitzer IRS Peak-Up imaging observations confirms the ex-tension also at16µm.This indicates that the10µm excess is of stellar origin,likely from the extended population of AGB stars.Renzini:One may expect that dust particled from AGB stars are destroyed by sputtering due to interaction with the hot,X-Ray emitting ISM of these galaxies.Is there any correlation of the strength of the emission with the X-ray luminosity of these galaxies? Bressan:We did not compare with the X-ray luminosity yet.However the dust distri-bution from stationary circumstellar envelopes is proportional to r−2and the emission is dominated by the innermost layers,where dust is likely not affected by interaction with the hot ISM.。
天文物理英文
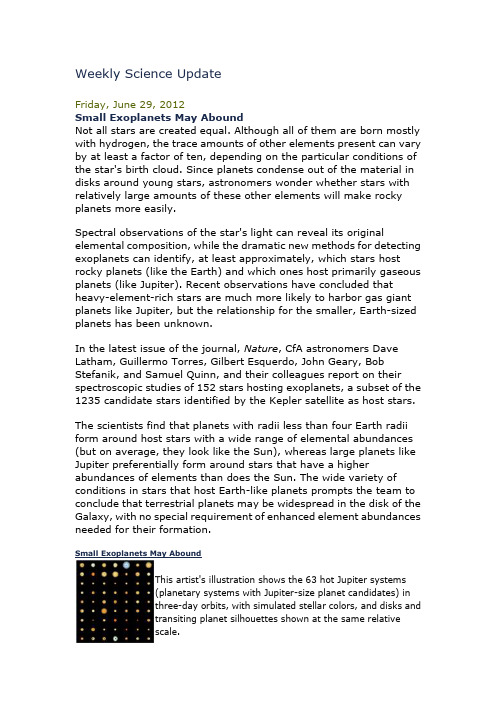
Weekly Science UpdateFriday, June 29, 2012Small Exoplanets May AboundNot all stars are created equal. Although all of them are born mostly with hydrogen, the trace amounts of other elements present can vary by at least a factor of ten, depending on the particular conditions of the star's birth cloud. Since planets condense out of the material in disks around young stars, astronomers wonder whether stars with relatively large amounts of these other elements will make rocky planets more easily.Spectral observations of the star's light can reveal its original elemental composition, while the dramatic new methods for detecting exoplanets can identify, at least approximately, which stars host rocky planets (like the Earth) and which ones host primarily gaseous planets (like Jupiter). Recent observations have concluded that heavy-element-rich stars are much more likely to harbor gas giant planets like Jupiter, but the relationship for the smaller, Earth-sized planets has been unknown.In the latest issue of the journal, Nature, CfA astronomers Dave Latham, Guillermo Torres, Gilbert Esquerdo, John Geary, Bob Stefanik, and Samuel Quinn, and their colleagues report on their spectroscopic studies of 152 stars hosting exoplanets, a subset of the 1235 candidate stars identified by the Kepler satellite as host stars. The scientists find that planets with radii less than four Earth radii form around host stars with a wide range of elemental abundances (but on average, they look like the Sun), whereas large planets like Jupiter preferentially form around stars that have a higher abundances of elements than does the Sun. The wide variety of conditions in stars that host Earth-like planets prompts the team to conclude that terrestrial planets may be widespread in the disk of the Galaxy, with no special requirement of enhanced element abundances needed for their formation.Small Exoplanets May AboundThis artist's illustration shows the 63 hot Jupiter systems(planetary systems with Jupiter-size planet candidates) inthree-day orbits, with simulated stellar colors, and disks andtransiting planet silhouettes shown at the same relativescale.Credit: Fermilab Center for Particle Astrophysics/J SteffenHigh Resolution Image (jpg)Low Resolution Image (jpg)Weekly Science UpdateWeekly Science UpdateRadio Galaxies in the Distant UniverseA small section from a Hubble image of distant galaxies. Anew paper has detected and studied infrared counterparts toall the galaxies in the main image with strong radio emission,the first time such a complete sample has been obtained. Credit: NASA/HubbleLow Resolution Image (jpg)Back to Science UpdateFriday, June 22, 2012Radio Galaxies in the Distant UniverseFor over a decade astronomers have been probing a region of the northern sky, not far from the handle of the Big Dipper, that is relatively free of bright stars and the diffuse glow of the Milky Way. The scientists want to take advantage of the clarity of the sky there to peer beyond our galaxy to study remote galaxies in the distant universe. This region, about half the angular size of the full moon, is now known to have over 50,000 galaxies.CfA astronomers Steve Willner, Matt Ashby, and Jia-Sheng Huang and their colleagues studied the region using the SAO-led Infrared Array Camera (IRAC) on the Spitzer Space Telescope. Surveys have detected 1122 galaxies in this region that emit strongly at radio wavelengths, a consequence of their undergoing active star formation or of hosting active supermassive black holes at their nuclei. Since the radio observations alone are unable to estimate the distances to the galaxies or unravel the precise mechanisms powering their emission, the team undertook to use infrared data to provide that information.In a paper to appear in the Astrophysical Journal, the team reports that it has detected essentially 100% of the radio galaxies in their infrared images. This is the first sample of the deep sky that has been able to completely associate radio galaxies with infrared counterparts, and it means that the conclusions they reach will be much more reliable. The team finds that 10-15% of the galaxies, most of them within a few billion light-years of us, are undergoing bursts of star formation. Roughly another quarter of the galaxies have supermassive black holes that are actively accreting matter; this group lies at greater distances (light from the most distant ones has been traveling for over eleven billion years). The remainder of the galaxies are still of uncertain nature, but now that both radio and infrared observations are available for all of them, future follow-up studies will have a strong basis for proceeding.。
StatementofResearchInterestsandGoals
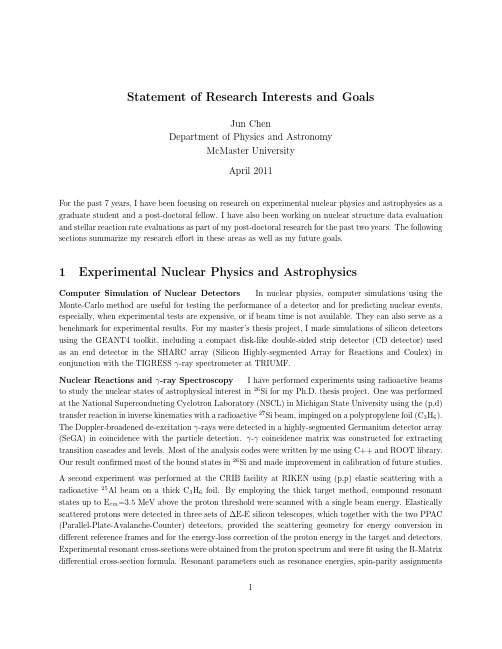
Statement of Research Interests and GoalsJun ChenDepartment of Physics and AstronomyMcMaster UniversityApril2011For the past7years,I have been focusing on research on experimental nuclear physics and astrophysics as a graduate student and a post-doctoral fellow.I have also been working on nuclear structure data evaluation and stellar reaction rate evaluations as part of my post-doctoral research for the past two years.The following sections summarize my research effort in these areas as well as my future goals.1Experimental Nuclear Physics and AstrophysicsComputer Simulation of Nuclear Detectors In nuclear physics,computer simulations using the Monte-Carlo method are useful for testing the performance of a detector and for predicting nuclear events, especially,when experimental tests are expensive,or if beam time is not available.They can also serve as a benchmark for experimental results.For my master’s thesis project,I made simulations of silicon detectors using the GEANT4toolkit,including a compact disk-like double-sided strip detector(CD detector)used as an end detector in the SHARC array(Silicon Highly-segmented Array for Reactions and Coulex)in conjunction with the TIGRESSγ-ray spectrometer at TRIUMF.Nuclear Reactions andγ-ray Spectroscopy I have performed experiments using radioactive beams to study the nuclear states of astrophysical interest in26Si for my Ph.D.thesis project.One was performed at the National Superconducting Cyclotron Laboratory(NSCL)in Michigan State University using the(p,d) transfer reaction in inverse kinematics with a radioactive27Si beam,impinged on a polypropylene foil(C3H6). The Doppler-broadened de-excitationγ-rays were detected in a highly-segmented Germanium detector array (SeGA)in coincidence with the particle detection.γ-γcoincidence matrix was constructed for extracting transition cascades and levels.Most of the analysis codes were written by me using C++and ROOT library. Our result confirmed most of the bound states in26Si and made improvement in calibration of future studies.A second experiment was performed at the CRIB facility at RIKEN using(p,p)elastic scattering with a radioactive25Al beam on a thick C3H6foil.By employing the thick target method,compound resonant states up to E cm=3.5MeV above the proton threshold were scanned with a single beam energy.Elastically scattered protons were detected in three sets of∆E-E silicon telescopes,which together with the two PPAC (Parallel-Plate-Avalanche-Counter)detectors,provided the scattering geometry for energy conversion in different reference frames and for the energy-loss correction of the proton energy in the target and detectors. Experimental resonant cross-sections were obtained from the proton spectrum and werefit using the R-Matrix differential cross-section formula.Resonant parameters such as resonance energies,spin-parity assignments1and widths were extracted from thisfit to the resonances that were prominent in the excitation function. Most of the program coding for the data analysis was written by me using C/C++and Fortran.Apart from my research projects,I have been also involved in other experiments such as direct measurements with radioactive ion beams at TRIUMF,and indirect measurements with transfer reactions and in-beam γ-ray spectroscopy at Yale University and the University of Tsukuba.Experimental Nuclear Astrophysics My interest is in stellar reaction rates of key reactions in explo-sive environments,such as in nova and supernova explosions.These reaction rates provide very important clues for understanding the nucleosynthesis in our galaxy.My PhD thesis project was on the25Al(p,γ)26Si reaction rate,which strongly influences the production of galactic26Al at nova temperatures.The goal was to reduce the existing large uncertainty in this rate,which therefore provides more accurate inputs for network calculations of nucleosynthesis in these environments.2Nuclear Structure Data EvaluationI have been working on nuclear structure data evaluation for the data project of the National Nuclear Data Center(NNDC)at Brookhaven National Laboratory(BNL)since2009.So far,I have completed evaluations on the nuclear structure of nuclei in the mass chains A=33,35,37(partial contribution)and44.In these evaluations,detailed evaluated level properties and related information are presented,including adopted values of level andγ-ray energies,decay data(energies,intensities and placement of radiations),and other spectroscopic data.I have also developed some computer programs which have been used to facilitate the evaluation process.3Stellar Reaction Rate EvaluationAs I am interested in stellar reaction rates,it was a natural step for me to do some evaluations of stellar reaction rates which are closely coupled to our group’s experimental program.This effort falls under the umbrella of the astrophysical reaction rate evaluation project at Oak Ridge National Laboratory(ORNL).I have evaluated and updated some important astrophysical reaction rates,such as the23Mg(p,γ)24Al which serves as a bridge between the NeNa cycle and the MgAl cycle in O-Ne classical novae,the29P(p,γ)30S which is a key reaction that affects the production and destruction of29Si and30Si in nova outbursts,and 25Al(p,γ)26Si.4Future GoalsI am very interested in experimental nuclear physics and nuclear astrophysics,particularly in nuclear struc-ture and astrophysical reaction rates.I have been involved in several experiments in such areas to date. There is still a large unknown territory to be explored.One of my goals is to study the nuclear structure of the nuclei in the astrophysically important reactions and their rates using improved experimental techniques and conditions.Another goal of mine is to evaluate and update the nuclear structure of additional mass chains,as well as new stellar reaction rates.2。
天文学专业词汇英汉对照
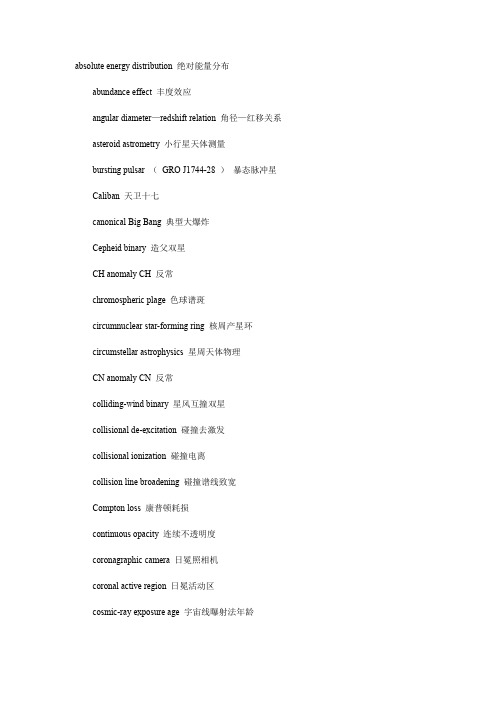
absolute energy distribution 绝对能量分布abundance effect 丰度效应angular diameter—redshift relation 角径—红移关系asteroid astrometry 小行星天体测量bursting pulsar (GRO J1744-28 )暴态脉冲星Caliban 天卫十七canonical Big Bang 典型大爆炸Cepheid binary 造父双星CH anomaly CH 反常chromospheric plage 色球谱斑circumnuclear star-forming ring 核周产星环circumstellar astrophysics 星周天体物理CN anomaly CN 反常colliding-wind binary 星风互撞双星collisional de-excitation 碰撞去激发collisional ionization 碰撞电离collision line broadening 碰撞谱线致宽Compton loss 康普顿耗损continuous opacity 连续不透明度coronagraphic camera 日冕照相机coronal active region 日冕活动区cosmic-ray exposure age 宇宙线曝射法年龄count—magnitude relation 计数—星等关系Cousins color system 卡曾斯颜色系统dating method 纪年法DDO color system DDO 颜色系统deep sky object 深空天体deep sky phenomena 深空天象dense star cluster 稠密星团diagnostics 诊断法dissociative recombination 离解复合Doppler line broadening 多普勒谱线致宽epicyclic orbit 本轮轨道extragalactic background 河外背景extragalactic background radiation 河外背景辐射flare particle emission 耀斑粒子发射flare physics 耀斑物理Fm star Fm 星focal plane spectrometer 焦面分光计focusing X-ray telescope 聚焦X 射线望远镜Friedmann time 弗里德曼时间galactic chimney 星系通道Galactic chimney 银河系通道gas relention age 气体变异法年龄Gauss line profile 高斯谱线轮廓GCR (Galactic cosmic rays )银河系宇宙线Geneva color system 日内瓦颜色系统global oscilletion 全球振荡GW-Vir instability strip 室女GW 不稳定带Highly Advanced Laboratory for 〈HALCA〉通讯和天文高新空间Communications and Astronomy 实验室(HALCA )Hipparcos catalogue 依巴谷星表Hobby-Eberly Telescope (HET )〈HET〉大型拼镶镜面望远镜Hoyle—Narlikar cosmology 霍伊尔—纳里卡宇宙学Hubble Deep Field (HDF )哈勃深空区human space flight 载人空间飞行、人上天imaging spectrograph 成象摄谱仪infrared camera 红外照相机infrared luminosity 红外光度infrared polarimetry 红外偏振测量in-situ acceleration 原位加速intercept age 截距法年龄inverse Compton limit 逆康普顿极限isochron age 等龄线法年龄Johnson color system 约翰逊颜色系统K giant variable (KGV )K 型巨变星kinetic equilibrium 运动学平衡large-scale beam 大尺度射束large-scale jet 大尺度喷流limb polarization 临边偏振line-profile variable 谱线轮廓变星long term fluctuation 长期起伏Lorentz line profile 洛伦兹谱线轮廓magnetic arm 磁臂Mars globe 火星仪massive black hole 大质量黑洞mean extinction coefficient 平均消光系数mean luminosity density 平均光度密度microwave storm 微波噪暴Milli-Meter Array (MMA )〈MMA〉毫米波射电望远镜阵molecular maser 分子微波激射、分子脉泽moving atmosphere 动态大气neutrino loss rate 中微子耗损率non-linear astronomy 非线性天文non-standard model 非标准模型passband width 带宽P Cygni type star 天鹅P 型星Perseus chimney 英仙通道planetary companion 似行星伴天体plateau phase 平台阶段primordial abundance 原始丰度protobinary system 原双星proto-brown dwarf 原褐矮星quiescent galaxy 宁静星系radiation transport 辐射转移radio-intermediate quasar 中介射电类星体random peculiar motion 随机本动relative energy distribution 相对能量分布RGU color system RGU 颜色系统ringed barred galaxy 有环棒旋星系ringed barred spiral galaxy 有环棒旋星系rise phase 上升阶段Rossi X-ray Timing Explorer (RXTE )〈RXTE〉X 射线时变探测器RQPNMLK color system RQPNMLK 颜色系统Scheuer—Readhead hypothesis 朔伊尔—里德黑德假说Serpens molecular cloud 巨蛇分子云soft X-ray transient (SXT )软X 射线暂现源solar dynamo 太阳发电机solar global parameter 太阳整体参数solar neighbourhood 太阳附近空间spectral catalogue 光谱表spectral duplicity 光谱成双性star-formation process 产星过程star-forming phase 产星阶段Stroemgren color system 颜色系统Sub-Millimeter Array (SMA )〈SMA〉亚毫米波射电望远镜阵superassociation 超级星协supermassive black hole 特大质量黑洞supersoft X-ray source 超软X 射线源super-star cluster 超级星团Sycorax 天卫十七symbiotic recurrent nova 共生再发新星synchrotron loss 同步加速耗损time dilation 时间扩展tired-light model 光线老化宇宙模型tremendous outburst amplitude 巨爆幅tremendous outburst amplitude dwarf 巨爆幅矮新星nova (TOAD )Tycho catalogue 第谷星表UBV color system UBV 颜色系统UBVRI color system UBVRI 颜色系统ultraviolet luminosity 紫外光度unrestricted orbit 无限制性轨道uvby color system uvby 颜色系统VBLUW color system VBLUW 颜色系统V enus globe 金星仪Vilnius color system 维尔纽斯颜色系统Virgo galaxy cluster 室女星系团VLBA (Very Long Baseline Array )〈VLBA〉甚长基线射电望远镜阵V oigt line profile 佛克特谱线轮廓VRI color system VRI 颜色系统Walraven color system 沃尔拉文颜色系统waning crescent 残月waning gibbous 亏凸月waxing crescent 娥眉月waxing gibbous 盈凸月WBVR color system WBVR 颜色系统Wood color system 伍德颜色系统zodiacal light photometry 黄道光测光11-year solar cycle 11 年太阳周αCygni variable 天津四型变星δDoradus variable 剑鱼δ型变星Vainu Bappu Observatory 巴普天文台variable-velocity star 视向速度变星vectorial astrometry 矢量天体测量vector-point diagram 矢点图V ega 〈维佳〉行星际探测器V ega phenomenon 织女星现象velocity variable 视向速度变星V enera 〈金星〉号行星际探测器very strong-lined giant, VSL giant 甚强线巨星very strong-lined star, VSL star 甚强线星video astronomy 录象天文viewfinder 寻星镜Viking 〈海盗〉号火星探测器virial coefficient 位力系数virial equilibrium 位力平衡virial radius 位力半径virial temperature 位力温度virtual phase CCD 虚相CCDvisible arm 可见臂visible component 可见子星visual star 光学星VLT, Very Large Telescope 甚大望远镜void 巨洞V ondrak method 冯德拉克方法V oyager 〈旅行者〉号行星际探测器VSOP, VLBI Space Observatory 空间甚长基线干涉测量Programme 天文台计划wave-front sensor 波前传感器weak-line T Tauri star 弱线金牛T 型星Wesselink mass 韦塞林克质量WET, Whole Earth Telescope 全球望远镜WHT, William Herschel Telescope 〈赫歇尔〉望远镜wide-angle eyepiece 广角目镜wide binary galaxy 远距双重星系wide visual binary 远距目视双星Wild Duck cluster (M 11 )野鸭星团Wind 〈风〉太阳风和地球外空磁层探测器WIRE, Wide-field Infrared Explorer 〈WIRE〉广角红外探测器WIYN Telescope, Wisconsin-Indiana- 〈WIYN〉望远镜Yale-NOAO TelescopeWR nebula, Wolf-Rayet nebula WR 星云Wyoming Infrared Telescope 怀俄明红外望远镜xenobiology 外空生物学XMM, X-ray Mirror Mission X 射线成象望远镜X-ray corona X 射线冕X-ray eclipse X 射线食X-ray halo X 射线晕XTE, X-ray Timing Explorer X 射线计时探测器yellow straggler 黄离散星Yohkoh 〈阳光〉太阳探测器young stellar object (YSO )年轻恒星体ZAHB, zero-age horizontal branch 零龄水平支Zanstra temperature 赞斯特拉温度ZZ Ceti star 鲸鱼ZZ 型星γ-ray burster (GRB )γ射线暴源γ-ray line γ谱线γ-ray line astronomy γ谱线天文γ-ray line emission γ谱线发射ζAurigae binary 御夫ζ型双星ζAurigae variable 御夫ζ型变星TAMS, terminal-age main sequence 终龄主序Taurus molecular cloud (TMC )金牛分子云TDT, terrestrial dynamical time 地球力学时television guider 电视导星器television-type detector 电视型探测器Tenma 〈天马〉X 射线天文卫星terrestrial reference system 地球参考系tetrad 四元基thermal background 热背景辐射thermal background radiation 热背景辐射thermal pulse 热脉冲thermonuclear runaway 热核暴涨thick-disk population 厚盘族thinned CCD 薄型CCDthird light 第三光源time-signal station 时号台timing age 计时年龄tomograph 三维结构图toner 调色剂torquetum 赤基黄道仪TRACE, Transition Region and Coronal 〈TRACE〉太阳过渡区和日冕Explorer 探测器tracker 跟踪器transfer efficiency 转移效率transition region line 过渡区谱线trans-Nepturnian object 海外天体Trapezium cluster 猎户四边形星团triad 三元基tri-dimensional spectroscopy 三维分光triquetum 三角仪tuning-fork diagram 音叉图turnoff age 拐点年龄turnoff mass 拐点质量two-dimensional photometry 二维测光two-dimensional spectroscopy 二维分光UKIRT, UK Infrared Telescope Facility 联合王国红外望远镜UKST, UK Schmidt Telescope 联合王国施密特望远镜ultracompact H Ⅱregion 超致密电离氢区ultradeep-field observation 特深天区观测ultraluminous galaxy 超高光度星系ultrametal-poor star 特贫金属星Ulysses 〈尤利西斯〉太阳探测器unseen component 未见子星upper tangent arc 上正切晕弧unnumbered asteroid 未编号小行星Uranian ring 天王星环Ursa Major group 大熊星群Ursa Minorids 小熊流星群Sagittarius dwarf 人马矮星系Sagittarius dwarf galaxy 人马矮星系Sagittarius galaxy 人马星系Saha equation 沙哈方程Sakigake 〈先驱〉空间探测器Saturn-crossing asteroid 越土小行星Saturnian ringlet 土星细环Saturnshine 土星反照scroll 卷滚Sculptor group 玉夫星系群Sculptor Supercluster 玉夫超星系团Sculptor void 玉夫巨洞secondary crater 次级陨击坑secondary resonance 次共振secular evolution 长期演化secular resonance 长期共振seeing management 视宁度控管segregation 层化selenogony 月球起源学separatrice 分界sequential estimation 序贯估计sequential processing 序贯处理serendipitous X-ray source 偶遇X 射线源serendipitous γ-ray source 偶遇γ射线源Serrurier truss 赛路里桁架shell galaxy 壳星系shepherd satellite 牧羊犬卫星shock temperature 激波温度silicon target vidicon 硅靶光导摄象管single-arc method 单弧法SIRTF, Space Infrared Telescope 空间红外望远镜Facilityslitless spectroscopy 无缝分光slit spectroscopy 有缝分光slow pulsar 慢转脉冲星SMM, Solar Maximum MIssion 太阳极大使者SMT, Submillimeter Telescope 亚毫米波望远镜SOFIA, Stratospheric Observatory for 〈索菲雅〉机载红外望远镜Infrared Astronomysoft γ-ray burst repeater 软γ暴复现源soft γrepeater (SGR )软γ射线复现源SOHO, Solar and Heliospheric 〈索贺〉太阳和太阳风层探测器Observatorysolar circle 太阳圈solar oscillation 太阳振荡solar pulsation 太阳脉动solar-radiation pressure 太阳辐射压solar-terrestrial environment 日地环境solitary 孤子性soliton star 孤子星South Galactic Cap 南银冠South Galactic Pole 南银极space density profile 空间密度轮廓space geodesy 空间大地测量space geodynamics 空间地球动力学Spacelab 空间实验室spatial mass segregation 空间质量分层speckle masking 斑点掩模speckle photometry 斑点测光speckle spectroscopy 斑点分光spectral comparator 比长仪spectrophotometric distance 分光光度距离spectrophotometric standard 分光光度标准星spectroscopic period 分光周期specular density 定向密度spherical dwarf 椭球矮星系spin evolution 自旋演化spin period 自旋周期spin phase 自旋相位spiral 旋涡星系spiral arm tracer 示臂天体Spoerer minimum 斯珀勒极小spotted star 富黑子恒星SST, Spectroscopic Survey Telescope 分光巡天望远镜standard radial-velocity star 视向速度标准星standard rotational-velocity star 自转速度标准星standard velocity star 视向速度标准星starburst 星暴starburst galaxy 星暴星系starburst nucleus 星暴star complex 恒星复合体star-formation activity 产星活动star-formation burst 产星暴star-formation efficiency (SFE )产星效率star-formation rate 产星率star-formation region 产星区star-forming region 产星区starpatch 星斑static property 静态特性statistical orbit-determination 统计定轨理论theorysteep-spectrum radio quasar 陡谱射电类星体stellar environment 恒星环境stellar halo 恒星晕stellar jet 恒星喷流stellar speedometer 恒星视向速度仪stellar seismology 星震学Stokes polarimetry 斯托克斯偏振测量strange attractor 奇异吸引体strange star 奇异星sub-arcsec radio astronomy 亚角秒射电天文学Subaru Telescope 昴星望远镜subcluster 次团subclustering 次成团subdwarf B star B 型亚矮星subdwarf O star O 型亚矮星subgiant branch 亚巨星支submilliarcsecond optical astrometry 亚毫角秒光波天体测量submillimeter astronomy 亚毫米波天文submillimeter observatory 亚毫米波天文台submillimeter photometry 亚毫米波测光submillimeter space astronomy 亚毫米波空间天文submillimeter telescope 亚毫米波望远镜submillisecond optical pulsar 亚毫秒光学脉冲星submillisecond pulsar 亚毫秒脉冲星submillisecond radio pulsar 亚毫秒射电脉冲星substellar object 亚恒星天体subsynchronism 亚同步subsynchronous rotation 亚同步自转Sunflower galaxy (M 63 )葵花星系sungrazer comet 掠日彗星supercluster 超星团; 超星系团supergalactic streamer 超星系流状结构supergiant molecular cloud (SGMC )超巨分子云superhump 长驼峰superhumper 长驼峰星supermaximum 长极大supernova rate 超新星频数、超新星出现率supernova shock 超新星激波superoutburst 长爆发superwind galaxy 超级风星系supporting system 支承系统surface activity 表面活动surface-brightness profile 面亮度轮廓surface-channel CCD 表面型CCDSU Ursae Majoris star 大熊SU 型星SW AS, Submillimeter Wave Astronomy 亚毫米波天文卫星Satallitesymbiotic binary 共生双星symbiotic Mira 共生刍藁symbiotic nova 共生新星synthetic-aperture radar 综合孔径雷达systemic velocity 质心速度radial pulsator 径向脉动星radial-velocity orbit 分光解radial-velocity reference star 视向速度参考星radial-velocity standard star 视向速度标准星radial-velocity survey 视向速度巡天radio arm 射电臂radio counterpart 射电对应体radio loud quasar 强射电类星体radio observation 射电观测radio picture 射电图radio pollution 射电污染radio supernova 射电超新星rapid burster 快暴源rapidly oscillating Ap star 快速振荡Ap 星readout 读出readout noise 读出噪声recycled pulsar 再生脉冲星reddened galaxy 红化星系reddened object 红化天体reddened quasar 红化类星体red horizontal branch (RHB )红水平分支red nebulous object (RNO )红色云状体Red Rectangle nebula 红矩形星云redshift survey 红移巡天red straggler 红离散星reflex motion 反映运动regression period 退行周期regular cluster 规则星团; 规则星系团relaxation effect 弛豫效应reset 清零resonance overlap theory 共振重叠理论return-beam tube 回束摄象管richness parameter 富度参数Ring nebula (M 57、NGC 6720 )环状星云ring-plane crossing 环面穿越Rosalind 天卫十三ROSAT, Roentgensatellit 〈ROSAT〉天文卫星Rosette Molecular Cloud (RMC )玫瑰分子云Rossby number 罗斯贝数rotating variable 自转变星rotational evolution 自转演化rotational inclination 自转轴倾角rotational modulation 自转调制rotational period 自转周期rotational phase 自转相位rotational pole 自转极rotational velocity 自转速度rotation frequency 自转频率rotation phase 自转相位rotation rate 自转速率rubber second 负闰秒rubidium-strontium dating 铷锶计年pan 摇镜头parry arc 彩晕弧partial-eclipse solution 偏食解particle astrophysics 粒子天体物理path of annularity 环食带path of totality 全食带PDS, photo-digitizing system、PDS、数字图象仪、photometric data system 测光数据仪penetrative convection 贯穿对流pentaprism test 五棱镜检验percolation 渗流periapse 近质心点periapse distance 近质心距periapsis distance 近拱距perigalactic distance 近银心距perigalacticon 近银心点perimartian 近火点period gap 周期空隙period-luminosity-colour relation 周光色关系PG 1159 star PG 1159 恒星photoflo 去渍剂photographic spectroscopy 照相分光photometric accuracy 测光精度photometric error 测光误差photometric night 测光夜photometric standard star 测光标准星photospheric abundance 光球丰度photospheric activity 光球活动photospheric line 光球谱线planetary biology 行星生物学planetary geology 行星地质学Pleiad 昴团星plerion 类蟹遗迹plerionic remnant 类蟹遗迹plerionic supernova remnant 类蟹超新星遗迹plumbicon 氧化铅光导摄象管pluton 类冥行星p-mode p 模、压力模pointimg accuracy 指向精度point spread function 点扩散函数polarimetric standard star 偏振标准星polarization standard star 偏振标准星polar-ring galaxy 极环星系Portia 天卫十二post AGB star AGB 后恒星post-core-collapse cluster 核心坍缩后星团post-coronal region 冕外区post-main-sequence star 主序后星post red-supergiant 红超巨后星post starburst galaxy 星暴后星系post T Tauri star 金牛T 后星potassium-argon dating 钾氩计年precataclysmic binary 激变前双星precataclysmic variable 激变前变星preceding limb 西边缘、前导边缘precessing-disk model 进动盘模型precession globe 岁差仪precession period 进动周期preflash 预照光pre-main-sequence spectroscopic 主序前分光双星binarypre-planetary disk 前行星盘pre-white dwarf 白矮前身星primary crater 初级陨击坑primordial binary 原始双星principle of mediocrity 折衷原则progenitor 前身星; 前身天体progenitor star 前身星projected density profile 投影密度轮廓proper-motion membership 自行成员星proper reference frame 固有参考架proper reference system 固有参考系proplyd 原行星盘proto-binary 原双星proto-cluster 原星团proto-cluster of galaxies 原星系团proto-earth 原地球proto-galactic cloud 原星系云proto-galactic object 原星系天体proto-Galaxy 原银河系proto-globular cluster 原球状星团proto-Jupiter 原木星proto-planet 原行星proto-planetary disk 原行星盘proto-planetary system 原行星系proto-shell star 原气壳星proto-sun 原太阳pseudo body-fixed system 准地固坐标系Puck 天卫十五pulsar time scale 脉冲星时标pulsation axis 脉动对称轴pulsation equation 脉动方程pulsation frequency 脉动频率pulsation phase 脉动阶段pulsation pole 脉动极pulse light curve 脉冲光变曲线pyrometry 高温测量QPO, quasi-periodic oscillation 似周期振荡quantum cosmology 量子宇宙学quantum universe 量子宇宙quasar astronomy 类星体天文quiescence 宁静态naked-eye variable star 肉眼变星naked T Tauri star 显露金牛T 型星narrow-line radio galaxy (NLRG )窄线射电星系Nasmyth spectrograph 内氏焦点摄谱仪natural reference frame 自然参考架natural refenence system 自然参考系natural seeing 自然视宁度near-contact binary 接近相接双星near-earth asteroid 近地小行星near-earth asteroid belt 近地小行星带near-earth comet 近地彗星NEO, near-earth object 近地天体neon nova 氖新星Nepturian ring 海王星环neutrino astrophysics 中微子天文NNTT, National New Technology Telescope国立新技术望远镜NOAO, National Optical Astronomical 国立光学天文台Observatoriesnocturnal 夜间定时仪nodal precession 交点进动nodal regression 交点退行non-destroy readout (NDRO )无破坏读出nonlinear infall mode 非线性下落模型nonlinear stability 非线性稳定性nonnucleated dwarf elliptical 无核矮椭圆星系nonnucleated dwarf galaxy 无核矮星系nonpotentiality 非势场性nonredundant masking 非过剩遮幅成象nonthermal radio halo 非热射电晕normal tail 正常彗尾North Galactic Cap 北银冠NOT, Nordic Optical Telescope 北欧光学望远镜nova rate 新星频数、新星出现率NTT, New Technology Telescope 新技术望远镜nucleated dwarf elliptical 有核矮椭圆星系nucleated dwarf galaxy 有核矮星系number density profile 数密度轮廓numbered asteroid 编号小行星oblique pulsator 斜脉动星observational cosmology 观测宇宙学observational dispersion 观测弥散度observational material 观测资料observing season 观测季occultation band 掩带O-Ne-Mg white dwarf 氧氖镁白矮星one-parameter method 单参数法on-line data handling 联机数据处理on-line filtering 联机滤波open cluster of galaxies 疏散星系团Ophelia 天卫七optical aperture-synthesis imaging 光波综合孔径成象optical arm 光学臂optical disk 光学盘optical light 可见光optical luminosity function 光学光度函数optically visible object 光学可见天体optical picture 光学图optical spectroscopy 光波分光orbital circularization 轨道圆化orbital eccentricity 轨道偏心率orbital evolution 轨道演化orbital frequency 轨道频率orbital inclination 轨道倾角orbit plane 轨道面order region 有序区organon parallacticon 星位尺Orion association 猎户星协orrery 太阳系仪orthogonal transformation 正交变换oscillation phase 振动相位outer asteroid belt 外小行星带outer-belt asteroid 外带小行星outer halo cluster 外晕族星团outside-eclipse variation 食外变光overshoot 超射OVV quasar, optically violently OVV 类星体variable quasar、optically violent variablevquasaroxygen sequence 氧序Kalman filter 卡尔曼滤波器KAO, Kuiper Air-borne Observatory 〈柯伊伯〉机载望远镜Keck ⅠTelescope 凯克Ⅰ望远镜Keck ⅡTelescope 凯克Ⅱ望远镜Kuiper belt 柯伊伯带Kuiper-belt object 柯伊伯带天体Kuiper disk 柯伊伯盘LAMOST, Large Multi-Object Fibre 大型多天体分光望远镜Spectroscopic TelescopeLaplacian plane 拉普拉斯平面late cluster 晚型星系团LBT, Large Binocular Telescope 〈LBT〉大型双筒望远镜lead oxide vidicon 氧化铅光导摄象管Leo Triplet 狮子三重星系LEST, Large Earth-based Solar 〈LEST〉大型地基太阳望远镜Telescopelevel-Ⅰcivilization Ⅰ级文明level-Ⅱcivilization Ⅱ级文明level-Ⅲcivilization Ⅲ级文明Leverrier ring 勒威耶环Liapunov characteristic number 李雅普诺夫特征数(LCN )light crown 轻冕玻璃light echo 回光light-gathering aperture 聚光孔径light pollution 光污染light sensation 光感line image sensor 线成象敏感器line locking 线锁line-ratio method 谱线比法Liner, low ionization nuclear 低电离核区emission-line regionline spread function 线扩散函数LMT, Large Millimeter Telescope 〈LMT〉大型毫米波望远镜local galaxy 局域星系local inertial frame 局域惯性架local inertial system 局域惯性系local object 局域天体local star 局域恒星look-up table (LUT )对照表low-mass X-ray binary 小质量X 射线双星low-metallicity cluster 低金属度星团;低金属度星系团low-resolution spectrograph 低分辨摄谱仪low-resolution spectroscopy 低分辨分光low - z 小红移luminosity mass 光度质量luminosity segregation 光度层化luminous blue variable 高光度蓝变星lunar atmosphere 月球大气lunar chiaroscuro 月相图Lunar Prospector 〈月球勘探者〉Ly-αforest 莱曼-α森林MACHO (massive compact halo 晕族大质量致密天体object )Magellan 〈麦哲伦〉金星探测器Magellan Telescope 〈麦哲伦〉望远镜magnetic canopy 磁蓬magnetic cataclysmic variable 磁激变变星magnetic curve 磁变曲线magnetic obliquity 磁夹角magnetic period 磁变周期magnetic phase 磁变相位magnitude range 星等范围main asteroid belt 主小行星带main-belt asteroid 主带小行星main resonance 主共振main-sequence band 主序带Mars-crossing asteroid 越火小行星Mars Pathfinder 火星探路者mass loss rate 质量损失率mass segregation 质量层化Mayall Telescope 梅奥尔望远镜Mclntosh classification 麦金托什分类McMullan camera 麦克马伦电子照相机mean motion resonance 平均运动共振membership of cluster of galaxies 星系团成员membership of star cluster 星团成员merge 并合merger 并合星系; 并合恒星merging galaxy 并合星系merging star 并合恒星mesogranulation 中米粒组织mesogranule 中米粒metallicity 金属度metallicity gradient 金属度梯度metal-poor cluster 贫金属星团metal-rich cluster 富金属星团MGS, Mars Global Surveyor 火星环球勘测者micro-arcsec astrometry 微角秒天体测量microchannel electron multiplier 微通道电子倍增管microflare 微耀斑microgravitational lens 微引力透镜microgravitational lensing 微引力透镜效应microturbulent velocity 微湍速度millimeter-wave astronomy 毫米波天文millisecond pulsar 毫秒脉冲星minimum mass 质量下限minimum variance 最小方差mixed-polarity magnetic field 极性混合磁场MMT, Multiple-Mirror Telescope 多镜面望远镜moderate-resolution spectrograph 中分辨摄谱仪moderate-resolution spectroscopy 中分辨分光modified isochrone method 改进等龄线法molecular outflow 外向分子流molecular shock 分子激波monolithic-mirror telescope 单镜面望远镜moom 行星环卫星moon-crossing asteroid 越月小行星morphological astronomy 形态天文morphology segregation 形态层化MSSSO, Mount Stromlo and Siding 斯特朗洛山和赛丁泉天文台Spring Observatorymultichannel astrometric photometer 多通道天测光度计(MAP )multi-object spectroscopy 多天体分光multiple-arc method 复弧法multiple redshift 多重红移multiple system 多重星系multi-wavelength astronomy 多波段天文multi-wavelength astrophysics 多波段天体物理Ida 艾达(小行星243号)IEH, International Extreme Ultraviolet 〈IEH〉国际极紫外飞行器HitchhikerIERS, International Earth Rotation 国际地球自转服务Serviceimage deconvolution 图象消旋image degradation 星象劣化image dissector 析象管image distoration 星象复原image photon counting system 成象光子计数系统image sharpening 星象增锐image spread 星象扩散度imaging polarimetry 成象偏振测量imaging spectrophotometry 成象分光光度测量immersed echelle 浸渍阶梯光栅impulsive solar flare 脉冲太阳耀斑infralateral arc 外侧晕弧infrared CCD 红外CCDinfrared corona 红外冕infrared helioseismology 红外日震学infrared index 红外infrared observatory 红外天文台infrared spectroscopy 红外分光initial earth 初始地球initial mass distribution 初始质量分布initial planet 初始行星initial star 初始恒星initial sun 初始太阳inner coma 内彗发inner halo cluster 内晕族星团integrability 可积性Integral Sign galaxy (UGC 3697 )积分号星系integrated diode array (IDA )集成二极管阵intensified CCD 增强CCDIntercosmos 〈国际宇宙〉天文卫星interline transfer 行间转移intermediate parent body 中间母体intermediate polar 中介偏振星international atomic time 国际原子时International Celestial Reference 国际天球参考系Frame (ICRF )intraday variation 快速变化intranetwork element 网内元intrinsic dispersion 内廪弥散度ion spot 离子斑IPCS, Image Photon Counting System 图象光子计数器IRIS, Infrared Imager / Spectrograph 红外成象器/摄谱仪IRPS, Infrared Photometer / Spectro- 红外光度计/分光计meterirregular cluster 不规则星团; 不规则星系团IRTF, NASA Infrared Telescope 〈IRTF〉美国宇航局红外Facility 望远镜IRTS, Infrared Telescope in Space 〈IRTS〉空间红外望远镜ISO, Infrared Space Observatory 〈ISO〉红外空间天文台isochrone method 等龄线法IUE, International Ultraviolet 〈IUE〉国际紫外探测器ExplorerJewel Box (NGC 4755 )宝盒星团Jovian magnetosphere 木星磁层Jovian ring 木星环Jovian ringlet 木星细环Jovian seismology 木震学jovicentric orbit 木心轨道J-type star J 型星Juliet 天卫十一Jupiter-crossing asteroid 越木小行星Galactic aggregate 银河星集Galactic astronomy 银河系天文Galactic bar 银河系棒galactic bar 星系棒galactic cannibalism 星系吞食galactic content 星系成分galactic merge 星系并合galactic pericentre 近银心点Galactocentric distance 银心距galaxy cluster 星系团Galle ring 伽勒环Galilean transformation 伽利略变换Galileo 〈伽利略〉木星探测器gas-dust complex 气尘复合体Genesis rock 创世岩Gemini Telescope 大型双子望远镜Geoalert, Geophysical Alert Broadcast 地球物理警报广播giant granulation 巨米粒组织giant granule 巨米粒giant radio pulse 巨射电脉冲Ginga 〈星系〉X 射线天文卫星Giotto 〈乔托〉空间探测器glassceramic 微晶玻璃glitch activity 自转突变活动global change 全球变化global sensitivity 全局灵敏度GMC, giant molecular cloud 巨分子云g-mode g 模、重力模gold spot 金斑病GONG, Global Oscillation Network 太阳全球振荡监测网GroupGPS, global positioning system 全球定位系统Granat 〈石榴〉号天文卫星grand design spiral 宏象旋涡星系gravitational astronomy 引力天文gravitational lensing 引力透镜效应gravitational micro-lensing 微引力透镜效应great attractor 巨引源Great Dark Spot 大暗斑Great White Spot 大白斑grism 棱栅GRO, Gamma-Ray Observatory γ射线天文台guidscope 导星镜GW Virginis star 室女GW 型星habitable planet 可居住行星Hakucho 〈天鹅〉X 射线天文卫星Hale Telescope 海尔望远镜halo dwarf 晕族矮星halo globular cluster 晕族球状星团Hanle effect 汉勒效应hard X-ray source 硬X 射线源Hay spot 哈伊斑HEAO, High-Energy Astronomical 〈HEAO〉高能天文台Observatoryheavy-element star 重元素星heiligenschein 灵光Helene 土卫十二helicity 螺度heliocentric radial velocity 日心视向速度heliomagnetosphere 日球磁层helioseismology 日震学helium abundance 氦丰度helium main-sequence 氦主序helium-strong star 强氦线星helium white dwarf 氦白矮星Helix galaxy (NGC 2685 )螺旋星系Herbig Ae star 赫比格Ae 型星Herbig Be star 赫比格Be 型星Herbig-Haro flow 赫比格-阿罗流Herbig-Haro shock wave 赫比格-阿罗激波hidden magnetic flux 隐磁流high-field pulsar 强磁场脉冲星highly polarized quasar (HPQ )高偏振类星体high-mass X-ray binary 大质量X 射线双星high-metallicity cluster 高金属度星团;高金属度星系团high-resolution spectrograph 高分辨摄谱仪high-resolution spectroscopy 高分辨分光high - z 大红移Hinotori 〈火鸟〉太阳探测器Hipparcos, High Precision Parallax 〈依巴谷〉卫星Collecting SatelliteHipparcos and Tycho Catalogues 〈依巴谷〉和〈第谷〉星表holographic grating 全息光栅Hooker Telescope 胡克望远镜host galaxy 寄主星系hot R Coronae Borealis star 高温北冕R 型星HST, Hubble Space Telescope 哈勃空间望远镜Hubble age 哈勃年龄Hubble distance 哈勃距离Hubble parameter 哈勃参数Hubble velocity 哈勃速度hump cepheid 驼峰造父变星Hyad 毕团星hybrid-chromosphere star 混合色球星hybrid star 混合大气星hydrogen-deficient star 缺氢星hydrogenous atmosphere 氢型大气hypergiant 特超巨星Eagle nebula (M 16 )鹰状星云earty cluster 早型星系团early earth 早期地球early planet 早期行星early-stage star 演化早期星early stellar evolution 恒星早期演化early sun 早期太阳earth-approaching asteroid 近地小行星earth-approaching comet 近地彗星earth-approaching object 近地天体earth-crossing asteroid 越地小行星earth-crossing comet 越地彗星earth-crossing object 越地天体earth orientation parameter 地球定向参数earth rotation parameter 地球自转参数eccentric-disk model 偏心盘模型effect of relaxation 弛豫效应Egg nebula (AFGL 2688 )蛋状星云electronographic photometry 电子照相测光elemental abundance 元素丰度elliptical 椭圆星系elliptical dwarf 椭圆矮星系emulated data 仿真数据emulation 仿真encounter-type orbit 交会型轨道enhanced network 增强网络equatorial rotational velocity 赤道自转速度equatorium 行星定位仪equipartition of kinetic energy 动能均分eruptive period 爆发周期Eskimo nebula (NGC 2392 )爱斯基摩星云estimated accuracy 估计精度estimation theory 估计理论EUVE, Extreme Ultraviolet Explorer 〈EUVE〉极紫外探测器Exclamation Mark galaxy 惊叹号星系Exosat 〈Exosat〉欧洲X 射线天文卫星extended Kalman filter 扩充卡尔曼滤波器extragalactic jet 河外喷流extragalactic radio astronomy 河外射电天文extrasolar planet 太阳系外行星extrasolar planetary system 太阳系外行星系extraterrestrial intelligence 地外智慧生物extreme helium star 极端氦星Fabry-Perot imaging spectrograph 法布里-珀罗成象摄谱仪Fabry-Perot interferometry 法布里-珀罗干涉测量Fabry-Perot spectrograph 法布里-珀罗摄谱仪face-on galaxy 正向星系face-on spiral 正向旋涡星系facility seeing 人为视宁度fall 见落陨星fast pulsar 快转脉冲星fat zero 胖零Fermi normal coordinate system 费米标准坐标系Fermi-Walker transportation 费米-沃克移动fibre spectroscopy 光纤分光field centre 场中心field galaxy 场星系field pulsar 场脉冲星filter photography 滤光片照相观测filter wheel 滤光片转盘find 发见陨星finder chart 证认图finderscope 寻星镜first-ascent giant branch 初升巨星支first giant branch 初升巨星支flare puff 耀斑喷焰flat field 平场flat field correction 平场改正flat fielding 平场处理flat-spectrum radio quasar 平谱射电类星体flux standard 流量标准星flux-tube dynamics 磁流管动力学f-mode f 模、基本模following limb 东边缘、后随边缘foreground galaxy 前景星系foreground galaxy cluster 前景星系团formal accuracy 形式精度Foucaultgram 傅科检验图样Foucault knife-edge test 傅科刀口检验fourth cosmic velocity 第四宇宙速度frame transfer 帧转移Fresnel lens 菲涅尔透镜fuzz 展云CAMC, Carlsberg Automatic Meridian 卡尔斯伯格自动子午环Circlecannibalism 吞食cannibalized galaxy 被吞星系cannibalizing galaxy 吞食星系。
Spectroscopic investigation on the interaction of salidroside
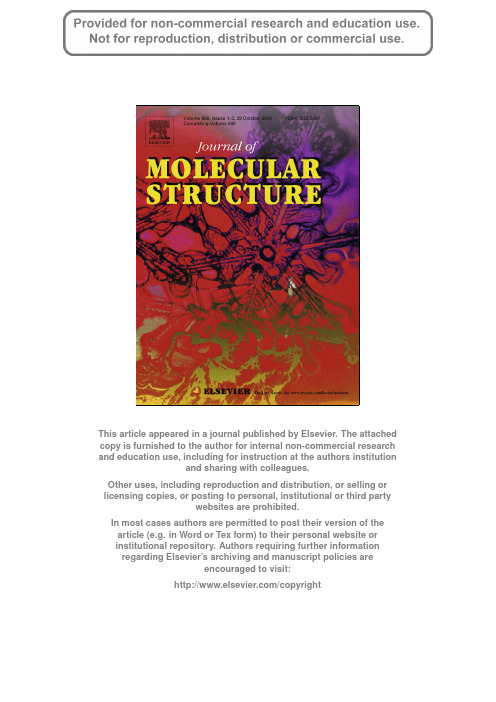
This article appeared in a journal published by Elsevier.The attached copy is furnished to the author for internal non-commercial research and education use,including for instruction at the authors institutionand sharing with colleagues.Other uses,including reproduction and distribution,or selling or licensing copies,or posting to personal,institutional or third partywebsites are prohibited.In most cases authors are permitted to post their version of thearticle(e.g.in Word or Tex form)to their personal website orinstitutional repository.Authors requiring further informationregarding Elsevier’s archiving and manuscript policies areencouraged to visit:/copyrightSpectroscopic investigation on the interaction of salidrosidewith bovine serum albuminZhengjun Cheng,Yuntao Zhang *Institute of Applied Chemistry,China West Normal University,Nanchong,Sichuan 637002,China Laboratory of Applied Chemistry and Pollution Control Technology,Nanchong,Sichuan,ChinaCollege of Chemistry and Chemical Engineering,China West Normal University,Nanchong,Sichuan 637002,ChinaReceived 18October 2007;received in revised form 19December 2007;accepted 7January 2008Available online 16January 2008AbstractThis study is designed to examine the interaction of salidroside with bovine serum albumin (BSA)under physiological conditions with drug concentrations in the range of 1.67–20.0l M.Spectroscopic analysis of the emission quenching at different temperatures has revealed that the quenching mechanism of salidroside with BSA is static quenching mechanism.The calculated distance r between sal-idroside and the protein is evaluated according to the theory of Forster energy transfer.The results of FTIR,CD,synchronous fluores-cence spectra and UV–vis absorption spectra experiment show that the secondary structures of the protein has been changed in the presence of salidroside.The thermodynamic parameters,enthalpy change (D H 0)and entropy change (D S 0)are calculated to be À50.50kJ mol À1and À59.13J mol À1K À1according to van’t Hoffequation,which indicate that the hydrogen bonds and van der Waals forces are the intermolecular force stabilizing the complex.The effects of common ions on the binding constants of BSA–salidroside com-plexes were also investigated.Ó2008Elsevier B.V.All rights reserved.Keywords:Bovine serum albumin;Salidroside;Fluorescence spectroscopy;FTIR spectroscopy;CD spectroscopy1.IntroductionSerum albumin as the major ligand binding and trans-port protein of circulatory system is considered as a model for studying drug–protein interaction in vitro [1].The most important property of this group of proteins is that they serve as a depot protein and as a transport protein for a variety of compounds [2].Bovine serum albumin (BSA)has been one of the most extensively studied of this group of proteins,particularly because of its structural homology (80%)with human serum albumin (HSA)[3].In view of this,we have planned to carry out the detailed investigations on the interaction of salidroside and BSA by using Fluorescence,FTIR,CD and UV–vis absorption spectroscopies techniques.Rhodiola sachalinensis A.Bor.(Hongjingtian in Chinese)is one of the most popular tradi-tional Chinese medicines.Salidroside is the main active compound in the root of Rhodiola sachalinensis.The con-centration of salidroside in Rhodiola has become one of the standard indexes to appraise the quality of Rhodiola [4].It is a phenolic glycoside and its chemical structure is shown in Fig.1.Recent studies have demonstrated that sal-idroside is a strong antioxidant and anticarcinogen [5].It has also been used in such special posts such as diver,astro-naut,pilot and mountaineer to enhance the body’s ability to survive in adverse environments [6].It has the activities of preventing cardiovascular disease and resisting cancers [7,8],extending human life [9].It can improve sleep and hypnotic action [10]and decrease the fat in blood [11].Sal-idroside has been found to be protective against neuron cell death induced by glutamate and hypoxia/hypoglycemia,and against mitochondria dysfunction induced by sodium azide [12].It protects SH-SY5Y cells from H 2O 2induced oxidative damage [12].The effectiveness of drugs depends0022-2860/$-see front matter Ó2008Elsevier B.V.All rights reserved.doi:10.1016/j.molstruc.2008.01.013*Corresponding author.Address:Institute of Applied Chemistry,China West Normal University,Nanchong,Sichuan 637002,China.Tel.:+868172314462;fax:+868172582029./locate/molstrucAvailable online at Journal of Molecular Structure 889(2008)20–27on their binding ability.So the studies on the binding of drugs to the protein may provide information of the struc-ture features that determine the therapeutic effectiveness of drugs.But,the mechanism of interaction of salidroside with BSA has not been studied in detail.In this paper,we have studied in vitro interaction of sal-idroside with BSA by the fluorescence quenching method.The effect of the energy transfer has been studied according to fluorescence resonance energy transfer.The binding con-stants are obtained at different temperatures in the medium of HCl–Tris (pH 7.4)buffer solution.The binding sites and main sorts of binding force have been suggested.In addi-tion,the conformational changes of BSA are discussed on the basis of synchronous fluorescence spectra,CD and FTIR.2.Experiment 2.1.MaterialsBovine serum albumin (BSA,FractionV)purchased from Sino-American Biotechnology Company,is used without further purification and its molecular weight is assumed to be 68000.All BSA solutions are prepared in the pH 7.4buffer solution,and BSA stock solution is kept in the dark at 4°C.Salidroside is of analytical grade and purchased from the National Institute for Control of Phar-maceutical and Products,China;NaCl (analytical grade,0.8mol L À1)is used to maintain the ion strength at 0.1.Buffer solution (pH 7.4)consists of Tris (0.1mol L À1)and HCl (0.1mol L À1),and the pH is adjusted to 7.4by adding 0.5mol L À1NaOH when the experiment tempera-ture is higher than 298K.All solutions are prepared with doubly distilled water.2.2.ApparatusThe fluorescence spectra are measured with an F-2500Spectrofluorimeter (Hitachi,Japan)equipped with a 150W Xenon lamp source and 1.0cm quartz cell and a thermostat bath.A Shimadzu UV-2550double-beam Spec-trophotometer (Shimadzu,Japan)equipped with 1.0cm quartz cells is used for scanning the UV spectrum at room temperature.Circular dichroism (CD)measurements are made on a Jasco-810automatic recording spectropolarimeter (Japan),using a 0.1cm cell at room temperature.The spectra are recorded in the range of 200–260nm and the scan rate is 100nm/min with a response time of 4s.CD determinations of pure BSA and BSA–salidroside complexes are carried out using the same solutions of salidroside at a correspond-ing concentration as the reference.The results are expressed as ellipticity (mdeg),which is obtained directly from the instrument.The content of a -helix is calculated from the following two equations:MRE ¼Observed CD ðmdeg ÞCnl Â10ð1Þa Àhelix ð%Þ¼ðÀMRE 208À4000Þ=ð33;000À4000Þf g Â100ð2Þwhere C is the molar concentration of the protein,n is the number of amino acid residues,l is the path length,MRE 208is the observed MRE value at 208nm.FT-IR measurements are carried out at 298K on a Brucker Equinox 55FT-IR spectrometer (Germany).All spectra are taken via the attenuated total reflection (ATR)method with resolution of 4cm À1and 32scans.Spectra processing procedures:the spectra of buffer solu-tion are collected under the same conditions.Then,sub-tract the absorbance of buffer solution from the spectra of sample solution to get the FTIR spectra of the protein.The subtraction criterion is that the original spectrum of the protein solution between 1800and 1300cm À1is a smooth straight.2.3.Spectroscopic measurementsThe absorption spectra of BSA,salidroside and their complexes are performed at room temperature and the fluorescence measurements are performed at different tem-peratures (298,305and 310K)in the range 300–500nm.BSA concentration is kept fixed at 5Â10À6mol L À1and salidroside concentrations are varied from 1.67to 20Â10À6mol L À1.The widths of both the excitation slit and the emission slit are set to 5.0nm.The excitation wave-length is 285nm and the emission wavelength is 347nm.Appropriate blanks corresponding to the buffer are sub-tracted to correct background fluorescence.3.Results and discussion3.1.Binding constants and fluorescence quenching mechanismIn this experiment,the concentrations of BSA are held constant at 5.0Â10À6mol L À1.The effect of salidroside on BSA fluorescence intensity is shown in Fig.2.As can be seen from Fig.2,addition of increasing concentrations of salidroside causes a decrease of the fluorescence inten-sity,but the maximum emission wavelength is hardly chan-ged.The result indicates that there is no change in the local dielectric environment.The different mechanisms of quenching are usually clas-sified as either dynamic quenching or staticquenching.Fig.1.Molecular structure of salidroside.Z.Cheng,Y.Zhang /Journal of Molecular Structure 889(2008)20–2721Dynamic and static quenching can be distinguished bytheir different dependence on temperature and viscosity.Dynamic quenching depends upon diffusion.Since higher temperatures result in larger diffusion coefficients,the bimolecular quenching constants are expected to increase with increasing temperature.In contrast,increasing tem-perature is likely to result in the decreased stability of com-plexes,and thus lower the values of the static quenching constants [13].The possible quenching mechanisms can be distinguished from each other with the Stern–Volmer plots shown in Fig.3.The results show that the Stern–Vol-mer plots are linear and the slopes decrease with increasing temperature.These plots imply static quenching between salidroside and BSA.In order to confirm this view,the data was first analyzed via assuming dynamic quenching to show that an unreasonable quenching constant will be obtained.The quenching equation is [14]:F 0F¼1þk q s 0½Q ¼1þK sv ½Q ð3Þwhere F 0and F are the fluorescence intensities in the absence and the presence of quencher,respectively;K sv ,k q ,s 0and [Q ]represent the Stern–Volmer quenching constant,the quenching rate constant of the biomolecule,the average lifetime of the protein without the quencher,the concentra-tion of the quencher (salidroside),respectively.The value of s 0of biopolymer is 10À8s À1[15].K sv is the slope of linearregressions of Fig.3.According to k q ¼K sv,the quenching constant k q can be calculated.The values of k q are of the order of 1012L mol À1s À1(Table 1).The maximum scatter collision quenching constant,k q of various quenching with the biopolymer is 2Â1010L mol À1s À1[16].Obviously,the constants k q of the protein quenching procedure initiated by salidroside are greater than the maximum scatter colli-sion quenching constant.This indicates that the quenching is not initiated by dynamic collision but from the formation of a complex.The static quenching equation is ðF 0ÀF ÞÀ1¼F À10þK À1a F À10½QÀ1ð4Þwhere K a is the binding constant of salidroside with the protein,which can be determined by the slope of the Lineweaver–Burk (1/(F 0ÀF )versus1/[Q ])curves as shown in Fig.4.Thus we can obtain binding constants K a of sal-idroside with BSA from the intercept and the slope of Fig.4(Table 2).The binding constants in Table 2indicate strong binding between BSA and salidroside and that the effect of temperature is small.As a result,the quenching of BSA by salidroside is not reduced significantly as tem-peratureincreases.Fig.2.Fluorescence spectra of BSA in the presence of various concen-trations of salidroside,c (BSA)=5.0l M;c (salidroside)/(l M),A–H:0;1.67;3.33;6.67;10.00;13.33;16.67;20.00.A concentration of 16.67l M(I)was used for salidrosideonly.Fig.3.The Stern–Volmer plot for the binding of salidroside with BSA at 298K (N ),305K ( ),310K (w ).Table 1The quenching rate constants between salidroside and BSA T (K)k q Â1012(L mol À1s À1)R 298 5.460.9984305 5.020.99793104.740.9964R is the correlationcoefficient.Fig.4.The Lineweaver–Burk plot for the binding of salidroside with BSA at 298K (s ),305K (M ),310K (h ).22Z.Cheng,Y.Zhang /Journal of Molecular Structure 889(2008)20–273.2.Binding parameters of BSA with salidrosideFor the static quenching interaction,if it is assumed that there are similar and independent binding sites in the bio-molecule,the binding constant (K )and the numbers sites (n )can be determined according to the method described by Wei et al.[17],using the equation:log F 0ÀF F ¼log K þn log ½Q ð5Þwhere K is the binding constant of salidroside with BSA and n is the number of binding site per albumin molecule,which were determined by the intercept and the slope ofdouble logarithm regression curves of log F 0ÀFF ÀÁversus log[Q ]based on the Eq.(5);the binding constants K and the number of binding sites n at pH 7.4can be ob-tained as K =4.51Â105L mol À1,n =1.21(R =0.9995)at 298K,K =1.68Â105L mol À1,n =1.12(R =0.9988)at 305K and K =0.43Â105L mol À1,n =0.98(R =0.9988)at 310K,respectively.The correlation coefficients are larger than 0.99,indicating that the assumptions under-lying the derivation of Eq.(5)are satisfied.The values of n at the experimental temperatures are approximately equal to 1,which indicates that there is one class of binding site to salidroside with BSA.In BSA,the trypto-phan residues involved in binding can be either Trp134or Trp212of both tryptophans in BSA,and Trp134is more exposed to a hydrophilic environment,whereas Trp212is deeply buried in the hydrophobic loop [18].So,from the value of n ,it is proposed that salidroside most likely bind to the hydrophobic pocket located in subdomain IIA.That is to say,Trp212is near or within the binding site [19].3.3.Determination of acting force between salidroside and BSAThe acting forces between a small molecule and macro-molecule include hydrogen bond,van der Waals force,elec-trostatic force,hydrophobic interaction force.The thermodynamic parameters,enthalpy change (D H 0),entropy change (D S 0)and free energy change (D G 0)are the main evidences to determine the binding mode.If the enthalpy changes do not vary significantly over the temper-ature range studied,then its value and D S 0value can be determined from the van’t Hoffand equation:log K a ¼ÀD H 02:303RT þD S 02:303Rð6ÞD G 0¼D H 0ÀT D S 0¼ÀRT ln K að7Þwhere K a and R are the binding constant and gas constant,respectively.The binding studies are carried out a 298,305and 310K.At these temperatures,BSA does not undergo any structural degradation.log K a versus 1/T plots enable the determination of D H 0,D S 0,D G 0and these values are summarized in Table 2.As can be seen in Table 2,the neg-ative values of D G 0,D H 0and D S 0are obtained for the sal-idroside–BSA interaction.Ross and Subramanian [20]have characterized the sign and magnitude of the thermo-dynamic parameter associated with various individual kinds of interaction that may take place in the protein asso-ciation processes,as described below.A positive D H 0value is frequently taken as typical evidence for hydrophobic interaction.Furthermore,specific electrostatic interactions between ionic species in aqueous solution are characterized by a positive D S 0and a negative D H 0value,while negative entropy and enthalpy changes arise from van der Waals forces and hydrogen bond formation in low dielectric med-ia.Accordingly,it is not possible to account for the ther-modynamic parameters of the salidroside–BSA coordination compound on the basis of a single intermolec-ular force model.Therefore,the negative values of both en-thalpy and entropy indicate that the binding of salidroside to BSA might involve both hydrogen bonding and van der Waals forces.3.4.Energy transfer between salidroside and BSAThe overlap of the UV absorption spectrum of salidro-side with the fluorescence emission spectrum of BSA is shown in Fig.5.According to Forster’s non-radiative energy transfer theory [21],the energy transfer will take place under these conditions:(1)the donor can produce fluorescent light that has sufficiently long lifetime;(2)the fluorescence emission spectrum of the donor and the UV absorbance spectrum of the acceptor have sufficient over-lap;(3)the distance between the donor and the acceptor is small (1–10nm)[22].The energy transfer effect is related not only to the distance between the acceptor and donor,but also to the critical energy transfer distance,that is E ¼R 60R 0þr6ð8ÞTable 2The binding constants K a and relative thermodynamic parameters of the salidroside–BSA system at different temperatures pH T (K)K a Â105(L mol À1)R a D G 0(kJ mol À1)D H 0(kJ mol À1)D S 0(J mol À1K À1)R b7.4298 5.780.9989À32.87À50.50À59.130.9997305 3.680.9991À32.503102.620.9994À32.16R a is the correlation coefficient for K a values.R b is the correlation coefficient for the van’t Hoffplot.Z.Cheng,Y.Zhang /Journal of Molecular Structure 889(2008)20–2723In Eq.(8),r represents the distance between donor and acceptor and R 0is the critical distance at which transfer efficiency equals to 50%.The value of R 0is calculated by using the equation [23],R 60¼8:8Â10À25k 2n À4/0J ð9Þwhere k is an orientation factor dependent on the align-ment of the donor and acceptor dipoles,n is the refractive index,and /0is the luminescence quantum yield in the ab-sence of energy transfer and J is the overlap between the luminescence spectrum of the donor and the absorption spectrum of the acceptor,J is given by [23]J ¼R 10F ðk ÞE A ðk Þk 4d k R 10F ðk Þd k ð10Þwhere F (k )is the corrected fluorescence intensity of the do-nor in the wavelength range k to k +D k and E A (k )is theextinction coefficient of the acceptor at k .The energy trans-fer efficiency is given by E ¼1ÀF F 0ð11Þwhere F and F 0are the fluorescence intensity of BSA in the presence and the absence of salidroside.From the overlapping spectrum of the absorption spec-tra of the acceptor and the fluorescence spectrum of the donor,J can be evaluated by integrating the spectra for k =300–500nm and J =5.44Â10À16cm À3L mol À1.Under these experimental calculations,we have found R 0=1.55nm from Eq.(9)by using k 2=2/3,n =1.36,/0=0.15for BSA [16],E =0.48and r =1.57nm from Eqs.(11)and (8)respectively.Obviously,the donor–accep-tor distance,r <8nm [25]indicates that the energy transfer from BSA to salidroside occurs with high possibility.These results accord with conditions of Forster’s non-radiativeenergy transfer theory,which indicates again the static quenching interaction between salidroside and BSA [24].3.5.UV–vis absorption studiesThe complex formation between salidroside–BSA is also evident from UV–vis absorption spectral data (Fig.6).As can be seen in Fig.6,the UV absorption intensity of BSA is increased with the addition of salidroside,and the maxi-mum peak positions of salidroside–BSA complexes are shifted slightly towards shorter wavelength,which indicates that it is due to complex formation between salidroside and BSA [16,24].3.6.Synchronous fluorescence spectroscopy studiesSynchronous fluorescence spectroscopy introduced by Llody [26]has been used to characterize complex mixtures providing fingerprints of complex samples [27].The Syn-chronous spectra are obtained by locking the excitation and emission monochromators drives together at a fixed increments of (D k )[26]or energy (D t )[28]difference.The resulting synchronous fluorescence spectra are simpler,narrower and hence more distinct than conventional exci-tation and emission fluorescence spectra [29].The synchro-nous fluorescence spectra only show the tyrosine residues and the tryptophan residues of BSA when the wavelength interval (D k )is 15and 60nm,respectively [30].The tyro-sine residues and the tryptophan residues of the fluores-cence spectra of BSA at various concentrations of salidroside are shown in Fig.7A and B,respectively.It is apparent from Fig.7that the maximum emission wavelength red shifts(from 348to 350.7nm)at the investi-gated concentrations range when D k =60nm,which is consistent with the fact that the conformation of BSAisFig.5.The overlap of the UV–vis absorption of salidroside with the fluorescence emission spectrum of BSA:(a)the UV–vis absorbance spectrum of salidroside,c (salidroside)=16.67l M and (b)the fluorescence spectrum of BSA,c (BSA)=5.0lM.Fig.6.Absorbance spectra of BSA,salidroside,and [BSA–salidroside complex]–[salidroside]system.BSA concentration is 1.0l M (a).Salidro-side concentration for [BSA–salidroside complex]–[salidroside]system is at 2.00l M (b),6.00l M (c),10.00l M (d).A concentration of 5.00l M (e)is used for salidroside only.24Z.Cheng,Y.Zhang /Journal of Molecular Structure 889(2008)20–27changed.It is also suggested a more polar (or less hydro-phobic)environment of tryptophan residue [13].3.7.CD spectra and FT-IR spectraTo obtain an insight into the structure of BSA,CD spec-tra are studied for the salidroside–BSA complex system.As Fig.8shows,the CD spectra of BSA exhibit two negative ellipticity in the UV region at 208and 220nm,characteris-tic of a -helix structures of the protein.The addition of sal-idroside causes a decrease in band intensity at all wavelengths of the far-UV CD,clearly indicating the decrease of the a -helix structures of the protein.This con-clusion agrees with the result of synchronous fluorescence spectroscopy experiment.However,the CD spectra of BSA in the absence and the presence of salidroside are sim-ilar in shape,indicating that the structure of BSA after the addition of salidroside is also predominantly a -helix.From the above results,it is apparent that the effects of salidro-side with BSA cause a conformational change of the pro-tein,with the loss of helical stability.From Eqs.(1)and (2),the a -helix contents of BSA are decreased from45.1%to 42.8%,38.4%,37.8%at molar rations of salidro-side to BSA of 0.32:1, 1.66:1, 3.2:1,respectively (c BSA =5.0l M).Fig.9is the FTIR spectra of the salidroside-free and sal-idroside-bound form of BSA with its difference absorption spectrum.The spectrum in Fig.9(A)is obtained by sub-tracting the absorption of the Tris–HCl from the spectrum of the protein solution.The spectrum in Fig.9(B)is obtained by subtracting the absorption of the salidroside-free form from that of the salidroside-bound form.The protein amide Ibands in the region 1600–1700cm À1(mainly C @O stretch)and amide II band %1548cm À1(C A N stretch coupled with N A H bending mode)both have a relationship with the secondary structure of the pro-tein.As shown in Fig.9,the peak position of amide I bands has not markedly changed but amide II moved from 1553.4to 1542.5cm À1,which indicates that the secondary struc-ture of the protein has been changed after salidroside is added.3.8.The effect of common ions on the binding constant of salidroside and BSAIn plasma,there are some metal ions,which can affect the reactions the drugs and the serum albumins.The effects of common ions,viz.,Zn 2+,Cu 2+,Ca 2+,Ba 2+,Ni 2+,CH 3COO Àand F Àon the binding constants of salidro-side–BSA system were investigated at 293K by recording the fluorescence intensity in the range 300–500nm excita-tion at 285nm and the adopted ions concentration are all 10times to the concentration of BSA.The results are summarized in Table 3.As evident from Table 3,the pres-ence of common ions reduced the binding constants of sal-idroside with BSA,causing salidroside to bequicklyFig.7.The synchronous fluorescence spectra of BSA at 15nm wavelength interval (A),at 60nm wavelength interval (B).In (A)and (B),the concentrations of BSA are kept fixed 5.0l M,and the concentrations of salidroside used are 0,1.67,5.00,11.67,15.00,18.33l M corresponding to the curves from top to bottom.(T =298K).Z.Cheng,Y.Zhang /Journal of Molecular Structure 889(2008)20–2725cleared from the blood,which may lead to need for more doses of salidroside to achieve the desired therapeutic effect [31].4.ConclusionsIn this paper,we have studied the interaction of BSA with salidroside by using many spectroscopic techniques. The results of CD,FTIR,synchronousfluorescence spectra and UV–vis spectra indicate that the secondary structure of the protein is changed in the presence of salidroside.The experimental results also indicate that a complex is formed between salidroside and BSA through the static quenching procedure.The thermodynamic parameters,enthalpy change(D H0)and entropy change(D S0)indicate that both hydrogen bonds and van der Waals forces play a role in the binding of salidroside to BSA.The binding constants of salidroside–BSA were decreased in the presence of com-mons ions.References[1]B.Ahmad,S.Parveen,R.H.Khan,Biomacromolecules7(2006)1350–1356.[2]A.Papadopoulou,R.J.Green,R.A.Frazier,J.Agric.Food Chem.53(2005)158–163.[3]A.Mallick,B.Haldar,N.Chattopadhyay,J.Phys.Chem.B109(2005)14683–14690.[4]X.Han,T.Y.Zhang,Y.Wei,X.L.Cao,Y.Ito,J.Chromatogr.A971(2002)237–241.[5]W.Zheng,S.Wang,J.Agric.Food Chem.49(2001)5165–5170.[6]H.B.Li,F.Chen,J.Chromatogr.A932(2001)91–95.[7]C.Z.Zhang,H.S.Yu,M.C.Lu,J.Li,F.X.Jin,Process Biochem.40(2005)3143–3147.[8]S.N.Udintsve,V.P.Schakhov,Eur.J.Cancer27(1991)1182.[9]V.A.Kurkin,J.Pharm.Chem.20(1986)1231–1235.[10]T.L.Li,G.H.Xu,L.L.Wu,C.Y.Sun,Phytomedicine14(2007)601–604.[11]R.W.Yang,G.Gao,T.Liu,S.L.Liu,G.X.Li,Electrochem.Commun.9(2007)94–96.[12]L.Zhang,H.X.Yu,Y.Sun,X.F.Lin,B.Chen,C.Tan,G.X.Cao,Z.W.Wang,Eur.J.Pharmacol.564(2007)18–25.[13]Y.J.Hu,Y.Liu,T.Q.Sun,A.M.Bai,J.Q.Lu,Z.B.Pi,Int.J.Biol.Macromol.39(2006)280–285.[14]Y.Q.Wang,H.M.Zhang,G.C.Zhang,W.H.Tao,S.H.Tang,J.Lumin.126(2007)211–218.[15]F.L.Cui,J.L.Wang,Y.R.Cui,J.P.Li,Anal.Chim.Acta571(2006)175–183.[16]P.B.Kandagal,S.Askoka,J.Seetharamappa,S.M.T.Shaikh,Y.Jadegoud,O.B.Ijare,J.Pharm.Biomed.Anal.41(2006)393–399.[17]Y.L.Wei,J.Q.Li,C.Dong,S.M.Shuang,D.S.Liu,C.W.Huie,Talanta70(2006)377–382.Table3The effects of common ions binding constant of salidroside–BSA systemSystem R Binding constant(Â104L molÀ1)BSA+salidroside0.9959 5.60BSA+salidroside+Zn2+0.9967 5.05BSA+salidroside+Cu2+0.9992 4.41BSA+salidroside+Ca2+0.9902 4.87BSA+salidroside+Ba2+0.9967 4.31BSA+salidroside+Ni2+0.9914 4.94BSA+salidroside+CH3COOÀ0.9901 3.57BSA+salidroside+FÀ0.9978 4.1726Z.Cheng,Y.Zhang/Journal of Molecular Structure889(2008)20–27[18]D.Subbaih,A.K.Mishra,J.Pharm.Biomed.Anal.38(2005)556–563.[19]A.Sulkowska,J.Mol.Struct.614(2002)227–232.[20]P.D.Ross,S.Subramanian,Biochemistry20(1981)3096–3102.[21]L.A.Sklar,B.S.Hudson,R.D.Simoni,Biochemistry16(1997)5100–5106.[22]G.W.Gordon,G.Berry,X.H.Liang,B.Levine,B.Herman,Biophys.J.74(1998)2702–2713.[23]A.Mahammed,H.B.Gray,J.J.Weaver,K.Sorasaenee,Z.Gross,Bioconjug.Chem.15(2004)738–746.[24]F.L.Cui,J.Fan,J.P.Li,Z.D.Hu,Bioorg.Med.Chem.12(2004)151–157.[25]B.Valeur,J.C.Brochon,New Trends in Fluorescence Spectroscopy,6th ed.,Springer Press,Berlin,1999,pp.25.[26]J.B.F.Llody,Nat.Phys.Sci.231(1971)64–65.[27]B.Apicella,A.Ciajolo,A.Tregrossi,Anal.Chem.76(2004)2138–2143.[28]E.L.Inman Jr.,J.D.Winefordner,Anal.Chem.54(1982)2018–2022.[29]T.Vo-Dinh,Anal.Chem.50(1978)396–401.[30]Y.P.Wang,Y.L.Wei,C.Dong,J.Photochem.Photobiol.A Chem.177(2006)6–11.[31]Y.Li,W.He,J.Liu,F.Sheng,Z.Hu,X.Chen,Biochim.Biophys.Acta1722(2005)15–21.Z.Cheng,Y.Zhang/Journal of Molecular Structure889(2008)20–2727。
The spectral energy distribution of self-gravitating protostellar disks
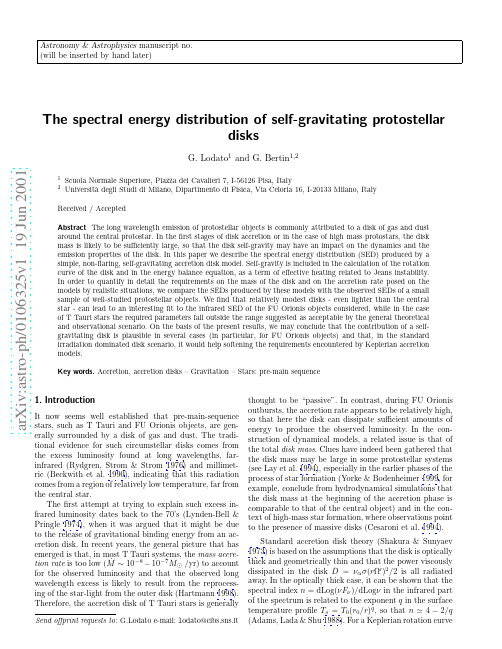
arXiv:astro-ph/01063
Scuola Normale Superiore, Piazza dei Cavalieri 7, I-56126 Pisa, Italy Universit` a degli Studi di Milano, Dipartimento di Fisica, Via Celoria 16, I-20133 Milano, Italy
Received / Accepted Abstract The long wavelength emission of protostellar objects is commonly attributed to a disk of gas and dust around the central protostar. In the first stages of disk accretion or in the case of high mass protostars, the disk mass is likely to be sufficiently large, so that the disk self-gravity may have an impact on the dynamics and the emission properties of the disk. In this paper we describe the spectral energy distribution (SED) produced by a simple, non-flaring, self-gravitating accretion disk model. Self-gravity is included in the calculation of the rotation curve of the disk and in the energy balance equation, as a term of effective heating related to Jeans instability. In order to quantify in detail the requirements on the mass of the disk and on the accretion rate posed on the models by realistic situations, we compare the SEDs produced by these models with the observed SEDs of a small sample of well-studied protostellar objects. We find that relatively modest disks - even lighter than the central star - can lead to an interesting fit to the infrared SED of the FU Orionis objects considered, while in the case of T Tauri stars the required parameters fall outside the range suggested as acceptable by the general theoretical and observational scenario. On the basis of the present results, we may conclude that the contribution of a selfgravitating disk is plausible in several cases (in particular, for FU Orionis objects) and that, in the standard irradiation dominated disk scenario, it would help softening the requirements encountered by Keplerian accretion models. Key words. Accretion, accretion disks – Gravitation – Stars: pre-main sequence
基于电子束离子阱的天体X射线和极紫外辐射研究进展
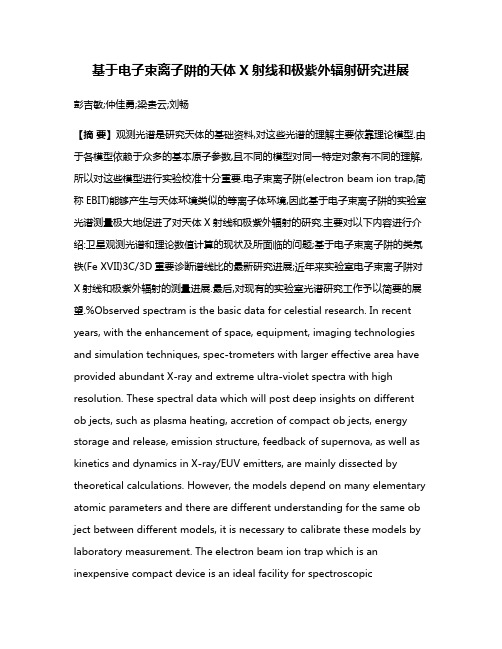
基于电子束离子阱的天体X射线和极紫外辐射研究进展彭吉敏;仲佳勇;梁贵云;刘畅【摘要】观测光谱是研究天体的基础资料,对这些光谱的理解主要依靠理论模型.由于各模型依赖于众多的基本原子参数,且不同的模型对同一特定对象有不同的理解,所以对这些模型进行实验校准十分重要.电子束离子阱(electron beam ion trap,简称EBIT)能够产生与天体环境类似的等离子体环境,因此基于电子束离子阱的实验室光谱测量极大地促进了对天体X射线和极紫外辐射的研究.主要对以下内容进行介绍:卫星观测光谱和理论数值计算的现状及所面临的问题;基于电子束离子阱的类氖铁(Fe XVII)3C/3D重要诊断谱线比的最新研究进展;近年来实验室电子束离子阱对X射线和极紫外辐射的测量进展.最后,对现有的实验室光谱研究工作予以简要的展望.%Observed spectram is the basic data for celestial research. In recent years, with the enhancement of space, equipment, imaging technologies and simulation techniques, spec-trometers with larger effective area have provided abundant X-ray and extreme ultra-violet spectra with high resolution. These spectral data which will post deep insights on different ob jects, such as plasma heating, accretion of compact ob jects, energy storage and release, emission structure, feedback of supernova, as well as kinetics and dynamics in X-ray/EUV emitters, are mainly dissected by theoretical calculations. However, the models depend on many elementary atomic parameters and there are different understanding for the same ob ject between different models, it is necessary to calibrate these models by laboratory measurement. The electron beam ion trap which is an inexpensive compact device is an ideal facility for spectroscopicbenchmark due to its overlapping electron density and temperature with as-trophysical plasma in stellar corona, supernova remnants and so on. Therefore, the spectral measurement based on the electron beam ion trap greatly promotes the study of X-ray and extreme ultraviolet radiation. This article will focus on the following: current situation of spectra of satellite observations and theoretical calculation and the problems; the latest re-search progress of the important diagnostic line ratio(Fe XVII's 3C/3D) based on EBIT;recent advances in laboratory measurement of X-rays and extreme ultraviolet radiation based on electron beam ion trap. Finally, a brief prospect for the laboratory spectroscopy research will be given.【期刊名称】《天文学进展》【年(卷),期】2017(035)004【总页数】12页(P417-428)【关键词】电子束离子阱;X射线;极紫外辐射;原子数据;高分辨率【作者】彭吉敏;仲佳勇;梁贵云;刘畅【作者单位】北京师范大学天文系,北京 100875;中国科学院光学天文重点实验室国家天文台,北京 100012;北京师范大学天文系,北京 100875;中国科学院光学天文重点实验室国家天文台,北京 100012;北京师范大学天文系,北京 100875【正文语种】中文【中图分类】P141了解天体辐射与光谱信息依赖于天体辐射成像和光谱观测,光谱获得主要基于高分辨率光谱仪观测。
地球化学_介绍 Geochemistry_Introduction
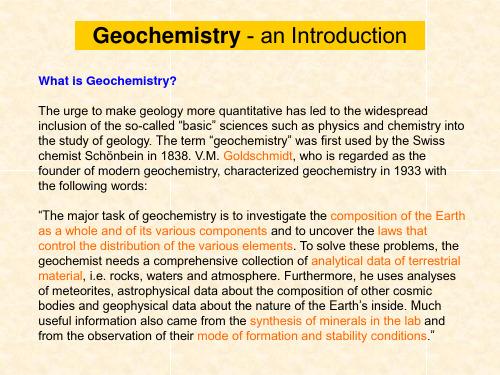
Definition and Sub-disciplines
Geochemistry uses the tools of chemistry to understand processes on Earth. The wide field of Geochemistry includes: Trace element geochemistry Isotope geochemistry Petrochemistry Soil geochemistry Sediment geochemistry Marine geochemistry Atmospheric geochemistry Planetary geochemistry Geochemical thermodynamics and kinetics Aquatic chemistry Inorganic geochemistry Organic geochemistry Biogeochemistry Environmental geochemistry …
Chemical Properties of the Elements
Pauling Scale The Pauling Scale is the most commonly used scale of electronegativity values. The calculations used to arrive at the numbers in the scale are complex. It is most common to simply know the results of those calculations. The scale is based on Fluorine having the largest electronegativity with a value of 4.0. The Francium atom is assigned the lowest electronegativity value at 0.7. All other values are located between these extremes. Examples: Li--1.0 Be--1.5 B--2.0 C--2.5 N--3.0 O--3.5 F--4.0. R.S. Mulliken (1934) proposed an electronegativity scale in which the electronegativity, χM is related to the electron affinity EAv (a measure of the tendency of an atom to form a negative species) and the ionization potential IEv (a measure of the tendency of an atom to form a positive species) by the equation: χM = (IEv + EAv)/2 The subscript v denotes a specific valence state. The Mulliken electronegativities are expressed directly in energy units, usually electron volts.
围绕繁星写一篇作文
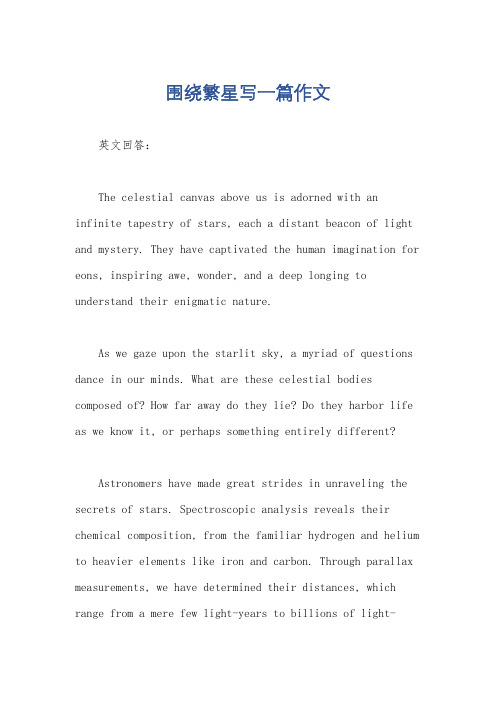
围绕繁星写一篇作文英文回答:The celestial canvas above us is adorned with aninfinite tapestry of stars, each a distant beacon of light and mystery. They have captivated the human imagination for eons, inspiring awe, wonder, and a deep longing to understand their enigmatic nature.As we gaze upon the starlit sky, a myriad of questions dance in our minds. What are these celestial bodies composed of? How far away do they lie? Do they harbor life as we know it, or perhaps something entirely different?Astronomers have made great strides in unraveling the secrets of stars. Spectroscopic analysis reveals their chemical composition, from the familiar hydrogen and helium to heavier elements like iron and carbon. Through parallax measurements, we have determined their distances, which range from a mere few light-years to billions of light-years away.The life cycles of stars are equally fascinating. They begin as clouds of gas and dust, known as nebulae. Gradually, under the influence of gravity, these clouds collapse, forming protostars. Nuclear fusion ignites in the core of the protostar, transforming it into a full-fledged star. The star's brightness and color are determined by its mass and temperature.Stars spend most of their lives on what is known as the main sequence. During this stable period, they fuse hydrogen into helium in their cores, releasing energy that radiates into space. Eventually, however, the hydrogen fuel in the core is exhausted. This signals the end of thestar's main-sequence life and the beginning of its evolutionary journey.Depending on the star's mass, it may evolve into different types of celestial objects. Low-mass stars, like our sun, swell into red giants as they exhaust their hydrogen fuel. They then shed their outer layers, formingplanetary nebulae. The exposed core becomes a white dwarf, a slowly cooling remnant of the star's former glory.Massive stars, on the other hand, have a more dramatic end. When their hydrogen fuel is depleted, they undergo a supernova explosion, expelling their outer layers into the surrounding space. The supernova remnant may leave behind a neutron star, a rapidly spinning, highly magnetized object, or even a black hole, a region of spacetime from which nothing can escape.The study of stars has not only deepened our understanding of the universe but also has profound implications for our own existence. Stars are the cosmic furnaces that produce the heavy elements essential for life on Earth. They are the remnants of ancient stellar explosions that enriched the interstellar medium with the building blocks of planets. And they are the constant companions of our planet, guiding travelers andilluminating the night sky with their celestial glow.中文回答:繁星点点,如天上撒落的碎银,点缀着辽阔的夜空,勾勒出壮美而神秘的图案。
蒲熠星的英语作文
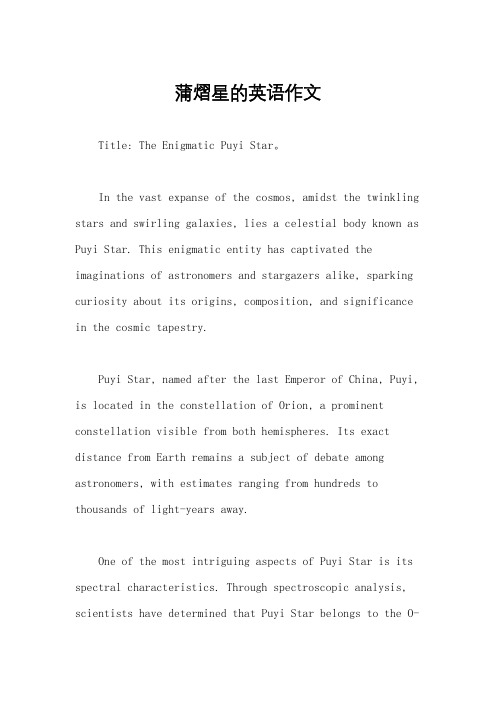
蒲熠星的英语作文Title: The Enigmatic Puyi Star。
In the vast expanse of the cosmos, amidst the twinkling stars and swirling galaxies, lies a celestial body known as Puyi Star. This enigmatic entity has captivated the imaginations of astronomers and stargazers alike, sparking curiosity about its origins, composition, and significance in the cosmic tapestry.Puyi Star, named after the last Emperor of China, Puyi, is located in the constellation of Orion, a prominent constellation visible from both hemispheres. Its exact distance from Earth remains a subject of debate among astronomers, with estimates ranging from hundreds to thousands of light-years away.One of the most intriguing aspects of Puyi Star is its spectral characteristics. Through spectroscopic analysis, scientists have determined that Puyi Star belongs to the O-type spectral class, indicating that it is a hot, massive, and luminous star. Its surface temperature surpasses 30,000 Kelvin, emitting intense ultraviolet radiation that ionizes the surrounding gas clouds, giving rise to dazzling nebulae.Moreover, Puyi Star exhibits peculiar variations in its luminosity, undergoing periodic fluctuations in brightness over time. Astronomers have observed irregular patterns in these variations, suggesting the presence of stellar companions or circumstellar disks that perturb its gravitational equilibrium.The composition of Puyi Star remains a subject of speculation, with astronomers proposing various theories to explain its origin and evolution. Some hypothesize that it emerged from a dense molecular cloud, undergoinggravitational collapse to form a protostellar disk from which Puyi Star and its planetary companions were born. Others suggest that it may be the product of a stellar merger or collision, resulting in the ejection of material and the formation of a new, more massive star.Furthermore, Puyi Star's role in the cosmic ecosystem extends beyond its mere existence. Its intense radiation influences the surrounding interstellar environment, sculpting vast regions of ionized gas and triggering the formation of new stars and planetary systems. Additionally, its eventual fate, whether it will culminate in a spectacular supernova explosion or evolve into a stable stellar remnant, remains a topic of speculation and intrigue.In conclusion, Puyi Star stands as a testament to the awe-inspiring complexity and beauty of the cosmos. Its enigmatic nature continues to challenge our understanding of stellar evolution and the mechanisms that govern the birth and death of stars. As we gaze upon the night sky and contemplate the mysteries of the universe, Puyi Star serves as a reminder of the boundless wonders that await our exploration and discovery.。
红外光谱和X-射线衍射表征水铁矿吸附砷酸的性质
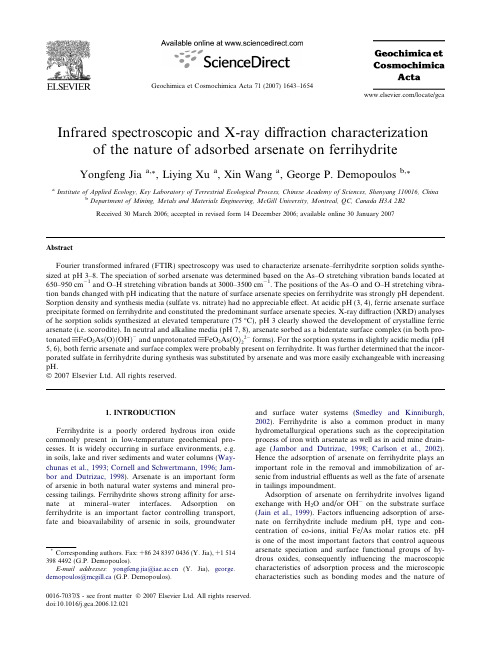
Infrared spectroscopic and X-ray diffraction characterizationof the nature of adsorbed arsenate on ferrihydriteYongfeng Jiaa,*,Liying Xu a ,Xin Wang a ,George P.Demopoulosb,*aInstitute of Applied Ecology,Key Laboratory of Terrestrial Ecological Process,Chinese Academy of Sciences,Shenyang 110016,ChinabDepartment of Mining,Metals and Materials Engineering,McGill University,Montreal,QC,Canada H3A 2B2Received 30March 2006;accepted in revised form 14December 2006;available online 30January 2007AbstractFourier transformed infrared (FTIR)spectroscopy was used to characterize arsenate–ferrihydrite sorption solids synthe-sized at pH 3–8.The speciation of sorbed arsenate was determined based on the As–O stretching vibration bands located at 650–950cm À1and O–H stretching vibration bands at 3000–3500cm À1.The positions of the As–O and O–H stretching vibra-tion bands changed with pH indicating that the nature of surface arsenate species on ferrihydrite was strongly pH dependent.Sorption density and synthesis media (sulfate vs.nitrate)had no appreciable effect.At acidic pH (3,4),ferric arsenate surface precipitate formed on ferrihydrite and constituted the predominant surface arsenate species.X-ray diffraction (XRD)analyses of he sorption solids synthesized at elevated temperature (75°C),pH 3clearly showed the development of crystalline ferric arsenate (i.e.scorodite).In neutral and alkaline media (pH 7,8),arsenate sorbed as a bidentate surface complex (in both pro-tonated B FeO 2As ðO ÞðOH ÞÀand unprotonated B FeO 2As ðO Þ22Àforms).For the sorption systems in slightly acidic media (pH 5,6),both ferric arsenate and surface complex were probably present on ferrihydrite.It was further determined that the incor-porated sulfate in ferrihydrite during synthesis was substituted by arsenate and was more easily exchangeable with increasing pH.Ó2007Elsevier Ltd.All rights reserved.1.INTRODUCTIONFerrihydrite is a poorly ordered hydrous iron oxide commonly present in low-temperature geochemical pro-cesses.It is widely occurring in surface environments,e.g.in soils,lake and river sediments and water columns (Way-chunas et al.,1993;Cornell and Schwertmann,1996;Jam-bor and Dutrizac,1998).Arsenate is an important form of arsenic in both natural water systems and mineral pro-cessing tailings.Ferrihydrite shows strong affinity for arse-nate at mineral–water interfaces.Adsorption on ferrihydrite is an important factor controlling transport,fate and bioavailability of arsenic in soils,groundwaterand surface water systems (Smedley and Kinniburgh,2002).Ferrihydrite is also a common product in many hydrometallurgical operations such as the coprecipitation process of iron with arsenate as well as in acid mine drain-age (Jambor and Dutrizac,1998;Carlson et al.,2002).Hence the adsorption of arsenate on ferrihydrite plays an important role in the removal and immobilization of ar-senic from industrial effluents as well as the fate of arsenate in tailings impoundment.Adsorption of arsenate on ferrihydrite involves ligand exchange with H 2O and/or OH Àon the substrate surface (Jain et al.,1999).Factors influencing adsorption of arse-nate on ferrihydrite include medium pH,type and con-centration of co-ions,initial Fe/As molar ratios etc.pH is one of the most important factors that control aqueous arsenate speciation and surface functional groups of hy-drous oxides,consequently influencing the macroscopic characteristics of adsorption process and the microscopic characteristics such as bonding modes and the nature of0016-7037/$-see front matter Ó2007Elsevier Ltd.All rights reserved.doi:10.1016/j.gca.2006.12.021*Corresponding authors.Fax:+862483970436(Y.Jia),+15143984492(G.P.Demopoulos).E-mail addresses:yongfeng.jia@ (Y.Jia),george.demopoulos@mcgill.ca (G.P.Demopoulos)./locate/gcaGeochimica et Cosmochimica Acta 71(2007)1643–1654adsorbed arsenate on the surface of ferrihydrite(Mas-scheleyn et al.,1991;Hsia et al.,1992;Fuller et al., 1993;Bowell,1994;Wilkie and Hering,1996;Raven et al.,1998;Jain et al.,1999;Meng et al.,2000;Grafe et al.,2002;Dixit and Hering,2003).The degree of pro-tonation of arsenate anion in aqueous solution is a func-tion of pH with p K a1=2.3,p K a2=6.8and p K a3=11.6 (Goldberg and Johnston,2001),resulting in arsenate spe-cies varying from H3AsO4,H2AsO4À,HAsO42À,toAsO43Àwhen pH increases from acidic region to alkalineregion(Myneni et al.,1998;Raven et al.,1998;Goldberg and Johnston,2001).On the other hand,the presence and the density of surface groups of ferrihydrite,i.e. H2O,OHÀ,are also strongly pH dependent.The point of zero charge(PZC)is approximately8.5(Jain et al., 1999;Goldberg and Johnston,2001).Hence,the modes of complexation of arsenate anions with ferrihydrite by replacing surface hydroxyl groups and/or waters are lar-gely controlled by the pH of reaction medium.Ligand exchange in the mode of bidentate binuclear in-ner-sphere complexation is the widely accepted mechanism of the adsorption of arsenate on iron oxides.It has been proposed based on infrared(Harrison and Berkheiser, 1982)and extended X-ray absorptionfine structure(EX-AFS)(Waychunas et al.,1993)analyses and confirmed to be the dominant interaction mode of arsenate–ferrihydrite and arsenate–goethite systems(Lumsdon et al.,1984;Man-ceau,1995;Sun and Doner,1996;Waychunas et al.,1996; Fendorf et al.,1997;Foster et al.,1998;Myneni et al.,1998; O’Reilly et al.,2001;Roddick-Lanzilotta et al.,2002;Sher-man and Randall,2003;Arai et al.,2004;Cance`s et al., 2005;Waychunas et al.,2005).However,most of the stud-ies were conducted at neutral to alkaline pH.The applica-bility of the conclusions to the acidic arsenate–ferrihydrite adsorption system may be questionable.Moreover,most of the studies have dealt mainly with characterizing the bonding mechanism between arsenate anions and surface iron polyhedra without identifying arsenate species on the surface of iron oxide.Based on macroscopic measurements of the adsorption process,arsenate was adsorbedas B FeO2AsðOHÞ2;B FeO2AsðOÞðOHÞÀ;B FeO2AsðOÞ22Àon the surface of ferrihydrite at mildly acidic and alkaline pH(Jain et al.,1999).In a recent work on direct character-ization of arsenate coordination on mineral(portlandite, gibbsite,ettringite,Fe-oxyhydroxides)surfaces using FTIR, both protonated and unprotonated arsenate species(i.e.HAsO42Àand AsO43Àwere present on the goethite surfaceat alkaline pH(Myneni et al.,1998).When arsenate was ad-sorbed on schwertmannite and ferrihydrite at acidic pH(i.e. pH3),surface precipitates were proposed to form and were termed as ferric hydroxyarsenate(FeOHAs)(Carlson et al., 2002).Evidence for surface precipitation of phosphate on goethite has been observed(Ler and Stanforth,2003).Sim-ilarly,surface precipitation of ferric arsenate on ferrihydrite is likely to occur in addition to bidentate binuclear com-plexation according to the XRD and Raman spectroscopic evidence we reported recently(Jia et al.,2006).The objective of this paper was to provide further evi-dence of surface precipitation of ferric arsenate on ferrihy-drite.This was done via characterization of the interactions between arsenate and ferrihydrite in terms of bonding modes and surface arsenate species as a function of pH and coverage density by Fourier transformed infrared spec-troscopy(FTIR)and evolution of crystallinity at elevated temperature(75°C)by X-ray diffraction(XRD)analysis. The effect of ferrihydrite synthesis media(NO3Àvs.SO42À)was also evaluated since it significantly influenced arsenate adsorption capacity(Jia and Demopoulos,2005). Poorly crystalline ferric arsenate was used as reference material in the study.Moreover,relatively high arsenic con-centration solutions were used in this study since this is the case in important hydrometallurgical operations where arsenic removal is practiced.2.MATERIALS AND METHODS2.1.Synthesis of poorly crystalline ferric arsenatePoorly crystalline ferric arsenate was synthesized at 21°C by adjusting a0.02M As(V)/0.02M Fe(III)solution (as sodium arsenate and ferric sulfate)from initial pH1.3to 1.8with NaOH solution and maintained at that pH for1h (Jia et al.,2006).The resultant solid was separated byfiltra-tion,washed with de-ionized water(pH2)and vacuum-dried at60°C.The chemical formula(Fe1.02AsO4Æ2.4H2O) was determined by digestion with hydrochloric acid fol-lowed by ICP-AES analysis.2.2.Synthesis of arsenate–ferrihydrite sorption samplesTwo-line ferrihydrite samples were synthesized at21°C using a slightly modified procedure from that reported in the literature(Schwertmann and Cornell,1991).Both ni-trate(Fe(NO3)3Æ9H2O)and sulfate(Fe2(SO4)3Æ5H2O)salts were used as the sources of ferric iron[Fe(III)].Briefly, the Fe(III)solution was prepared by dissolving ferric ni-trate or ferric sulfate in de-ionized water.The pH of the solution was raised to$7.5in about5min using1M NaOH solution and maintained at that pH for1h with the slurry mechanically agitated vigorously.The ferrihy-drite samples synthesized from sulfate and nitrate media were termed as‘‘sulfate–ferrihydrite’’and‘‘nitrate–ferrihy-drite’’,respectively,for simplicity.The prepared ferrihydrite slurry was adjusted to differ-ent pH between3and8with NaOH and HNO3and al-lowed to equilibrate for1h.Arsenate solution was introduced into the ferrihydrite slurry from a burette over a10-min period with the slurry mechanically stirred moder-ately.The pH was controlled by addition of NaOH and/or HNO3solution and allowed to equilibrate at21°C for 2weeks.The volume of adsorption slurry was500mL for all experiments and the concentration of Fe(III)in the slur-ry system was4g/L.At each pH,three initial Fe/As molar ratios(i.e.Fe/As=2,4and8)were applied.Arsenate–fer-rihydrite sorption samples were also synthesized at75°C, Fe/As molar ratio of2and4.The pH of the slurry was con-trolled constant at pH3throughout the sorption reaction. Samples were taken at1day,3day,1week,2weeks and 2months of reaction time.The synthesized arsenate–fer-rihydrite sorption products werefiltered,DI water-rinsed1644Y.Jia et al./Geochimica et Cosmochimica Acta71(2007)1643–1654and vacuum-dried at60°C.The equilibrium concentration of arsenic was determined by ICP-AES analysis.2.3.FTIR analysisThe infrared spectra of the samples were obtained on a Bio-Rad FTS60Fourier Transformed Infrared Spectrome-ter with a MCT liquid nitrogen cooled detector.The KBr/ sample discs were prepared by mixing0.5%offinely ground samples in KBr.The sample chamber was purged by N2gas for10min before scans were started.The measurement res-olution was set at4cmÀ1and the spectra were collected in the range of400–4000cmÀ1with200co-added scans.2.4.X-ray diffraction(XRD)analysisThe powder XRD patterns were collected on a Rigaku D/Max2500PC X-ray diffractometer with graphite mono-chromated CuK a1radiation.The powder samples were scanned from10to90°2h with increments of0.02°2h.3.RESULTS AND DISCUSSION3.1.Effect of pH on the nature of adsorbed arsenateAqueous arsenate species have no direct bearing on the surface arsenate species adsorbed on mineral surfaces (Myneni et al.,1998).However,the effect of complexation of arsenate ions on oxide surfaces is similar to that of pro-tonation of aqueous arsenate species.A brief discussion on infrared absorption of aqueous arsenate can assist with understanding the infrared characteristics of surface species (Myneni et al.,1998;Goldberg and Johnston,2001;Rod-dick-Lanzilotta et al.,2002).The free arsenate anion,AsO43À,is present in highly alkaline(p K a3=11.6)aqueoussolution and belongs to T d symmetry.Only m3and m4funda-mental bands are infrared active in this form.The infrared spectrum of an AsO43Àdominated solution exhibits a major band at792cmÀ1(Roddick-Lanzilotta et al.,2002).Uponprotonation or complexation with metal cations,the sym-metry decreases and splitting of the m3band occurs(Harri-son and Berkheiser,1982;Myneni et al.,1998).AqueousHAsO42Àspecies belong to the C3v symmetry.Its infrared spectrum shows two broad bands at859and689cmÀ1, the latter was assigned to stretching vibration of As–OH (Myneni et al.,1998).Curvefitting of the former band gave two bands at865and846cmÀ1,which were assigned to asymmetric and symmetric stretching vibration of uncom-plexed As–O,respectively(Myneni et al.,1998).Poorly crystalline ferric arsenate was used as reference material in this study to identify the possible occurrence of a surface precipitate of arsenate on ferrihydrite.This compound is not well defined and often termed loosely as amorphous ferric arsenate(Krause and Ettel,1989)or amorphous scorodite(Langmuir et al.,1999),because it possesses similar bonding structures to crystalline ferric arsenate,i.e.scorodite(FeAsO4Æ2H2O).It is an unstable arsenate phase with increasing pH and tends to convert to ferrihydrite(Krause and Ettel,1989).The poorly crystalline ferric arsenate synthesized in this work was determined to have the formula Fe1.02AsO4Æ2.4H2O.Fig.1shows the effect of pH on the infrared spectra of the sorption samples of arsenate on sulfate–ferrihydrite(initial molar ratio of Fe/As=2).Both detailed display of the As–O stretching vibration region(500–1000cmÀ1)and the whole range of the scanning(400–4000cmÀ1)are shown in thefigure.Poorly crystalline ferric arsenate shows a strong well-resolved band at838cmÀ1.Within the crystalline ferric arsenate(i.e.scorodite)structure,AsO4tetrahedra and FeO4(OH2)2octahedra connect alternately at vertices(Kita-hama et al.,1975).The arsenate is coordinated with four iron octahedra with an average As–O bond length of1.68A˚.The band at838cmÀ1was attributed to the stretching vibration of As–O coordinating to iron atom,i.e.As–O–Fe.The weakThe nature of adsorbed arsenate on ferrihydrite1645shoulder at$750cmÀ1was probably caused by the hydrogen bonding between H2O and AsO4since the H-bonding re-sulted in increased bond length and a red shift of the wave number(Myneni et al.,1998).The1625cmÀ1band was due to water O–H bending mode whereas the stretching vibration bands of O–H were located at$3194and$3373cmÀ1.The bands between950and1250cmÀ1were assigned to struc-tural SO42Àions,which were incorporated into the poorlycrystalline ferric arsenate by substitution of AsO43Àions dur-ing synthesis from sulfate medium.Sulfate ions were incorpo-rated into crystalline scorodite synthesized from sulfate solution(Singhania et al.,2005).The infrared spectra of pH3and4sorption samples also exhibited a strong,well-resolved band in the As–O stretching vibration region at similar position($833cmÀ1)to that of poorly crystalline ferric arsenate,indicating similarities of the arsenate bonding structures between sorption samples and poorly crystalline ferric arsenate.This suggested the for-mation of a ferric arsenate surface precipitate in the arsenate–ferrihydrite sorption samples synthesized in acidic media. However,the weak shoulder at$750cmÀ1on the FTIR spec-trum of the poorly crystalline ferric arsenate was missing for the sorption solids for some unknown reasons.The band at$833cmÀ1for the pH3and4sorption samples was assigned to As–O stretching vibration of the As–O–Fe coordination of ferric arsenate precipitate on fer-rihydrite.The formation of ferric arsenate phase in pH3 and4sorption samples was also supported by the O–H stretching vibration band at$3190cmÀ1.All samples showed a strong broad O–H band at$3370,but only theacidic sorption samples displayed the$3190cmÀ1O–H stretching vibration band like the case of poorly crystalline ferric arsenate.This characteristic O–H band of poorly crystalline ferric arsenate at$3190cmÀ1was fading out with increasing pH,indicating the disappearance of ferric arsenate surface precipitate at neutral and alkaline pH.As pH increased from3to8,the As–O stretching vibra-tion band shifted gradually from$833cmÀ1down to $806cmÀ1.At the same time,a new band emerged at high-er frequency(870–880cmÀ1)and its intensity was more pronounced with increasing pH.At pH8,we could clearly see the splitting of the single band into two bands.Peak deconvolution and curvefitting of the band produced two peaks at$806–810and$878cmÀ1(Fig.2).It is well estab-lished that at mildly alkaline pH,arsenate is adsorbed on ferrihydrite via bidentate binuclear complexation with surface iron polyhedra(Harrison and Berkheiser,1982; Waychunas et al.,1993).The band at$878cmÀ1of the pH6–8sorption samples was assigned to uncomplexed/ unprotonated As–O,whereas the$806–808cmÀ1arose from the two As–O–Fe complexed to ferrihydrite surface. Two infrared bands at824/861and817/854cmÀ1were ob-served for the arsenate adsorbed on amorphous iron oxide at pH5and9,respectively(Goldberg and Johnston,2001). The lower frequency bands at817and824cmÀ1were as-signed to the stretching vibration of As–O–Fe and the high-er frequency bands at854and861cmÀ1were attributed to ‘‘non-surface-complexed’’As–O bonds of the adsorbed arsenate species(Goldberg and Johnston,2001).It is inter-esting to note that the lower frequency band increased from 817and824cmÀ1as pH increased from5to9.This obser-vation and the band assignments are in good agreement with present work.Roddick-Lanzilotta et al.(2002)also reported that the As–O stretching vibration band of the ad-sorbed arsenate on ferrihydrite shifted from$825to $800cmÀ1as pH increased from2.6to8.In the case of bidentate binuclear complexation,two of the four As–O bonding structures are complexed to iron atoms(i.e.As–O–Fe)and the remaining two are present either both as unprotonated As–O or one as unprotonated As–O and the other one as protonated As–O–H.In com-parison,arsenate ions are coordinated to four iron atoms in ferric arsenate.According to Myneni et al.(1998),the force constant of the As–OM bond increases with coordina-tion number and decreases compared to uncomplexed As–O.Hence,for the bidentate adsorbed arsenate ion,the force constant of the two coordinated As–O–Fe is lower than that of the As–O–Fe in ferric arsenate,whereas the uncomplexed/unprotonated As–O bond has larger force constant compared to ferric arsenate.Consequently,the stretching vibration frequency of the uncomplexed/unprot-onated As–O is located at higher position while the fre-quency of the complexed As–O–Fe band is located at lower position.The increase and decrease of the As–O force constant for the bidentate binuclear complexed arsenate compared to ferric arsenate is supported by the As–O bond length(two at1.62,1.67A˚and the other two at1.71A˚, compared to1.68A˚of ferric arsenate)(Sherman and Ran-dall,2003).The shorter bond distance results in a stronger force constant and consequently higher infrared frequency.1646Y.Jia et al./Geochimica et Cosmochimica Acta71(2007)1643–1654A very weak band was observed at$700cmÀ1on the infrared spectrum of pH8arsenate–ferrihydrite sorption sample(see Fig.1).This band was reasonably assigned to protonated As–O–H bond of the adsorbed arsenate species, which was located at similar position to that of aqueous protonated arsenate species(Myneni et al.,1998).Complex-ation with metals cannot give such a low As–O stretching vibration frequency.The presence of protonated arsenate species was also proposed for the adsorption of arsenate on freshly prepared hydrous iron oxide and goethite(Myn-eni et al.,1998).It was noted that the weak As–O–H band at$700cmÀ1was absent at acidic pH indicating the ab-sence of protonated adsorbed arsenate species on ferrihy-drite at acidic pH.In a previous study using a dispersion infrared instrument(Harrison and Berkheiser,1982),three bands at875,805and700cmÀ1were observed for the ad-sorbed arsenate on hydrous ferric oxide(HFO)at pH6.5. They are very similar to the infrared bands of the pH8 sorption samples of this work(878,806and700cmÀ1).pH3and8are the extreme cases for the adsorption of arsenate on ferrihydrite in this work.At pH3,a sur-face precipitate developed and the adsorbed arsenate spe-cies were present mainly as poorly crystalline ferric arsenate.The possibility of surface precipitation of arse-nate on ferrihydrite was also suggested previously by Stanforth(1999)and Carlson et al.(2002).According to the latter research,a poorly crystalline ferric hydroxy-arsenate(FeOHAs)surface precipitate was found to form during adsorption of arsenate on schwertmannite and fer-rihydrite at pH3(Carlson et al.,2002).Similarly,surface precipitation of phosphate on goethite has been proposed in recent studies(Zhao and Stanforth,2001;Ler and Stanforth,2003).It was suggested that the adsorption reaction consisted of two phases:thefirst phase of rapid surface complexation followed by the second phase of slow buildup of a surface precipitate(Zhao and Stan-forth,2001).At the other extreme(i.e.pH8)arsenate was adsorbed via bidentate binuclear complexation with surface iron atoms in the form of unprotonated and probably protonated arsenate species as well(i.e.B FeO2AsðOÞ22Àand B FeO2AsðOÞðOHÞÀ, where B Fe represents the surface of ferrihydrite).This is in good agreement with the model proposed previously by Myneni et al.(1998),Jain et al.(1999)and Goldberg and Johnston(2001).Myneni et al.(1998)suggested that both protonated and unprotonated arsenate species were present as surface arsenate species adsorbed on ferrihydrite at alkaline pH.The surface arsenate specieswere proposed to be XH2AsO4,XHAsO4À,XAsO42À(X was Al or Fe)(Jain et al.,1999;Goldberg and Johnston, 2001).As pH increased from3to8,surface arsenate species shifted from poorly crystalline ferric arsenate precipitates to bidentate surface complexes.This is reasonable since poorly crystalline ferric arsenate is stable only at acidic pH and decomposes with increasing pH(Krause and Ettel, 1989).For the sorption systems whose media pHs lay be-tween3and8,both types of arsenate species were probably present on the surface of ferrihydrite,with the feature of poorly crystalline ferric arsenate being more pronounced at lower pH and the feature of bidentate complexes being more pronounced at higher pH.Similar to the Fe/As=2systems,the single As–O stretching vibration band shifted down gradually and split into two bands as pH increased from3to8for the Fe/As= 4sorption samples(Fig.3).The single band at acidic pH was assigned to As–O–Fe of ferric arsenate surface pre-cipitate.The presence of$3190cmÀ1O–H stretching vibra-tion band also indicated the development of ferric arsenate in the sorption solids at acidic pH.This band was absent onThe nature of adsorbed arsenate on ferrihydrite1647the infrared spectra of sorption samples at alkaline pH.The two bands(808and878cmÀ1)at alkaline pH are attributed to As–O–Fe bidentate–binuclear coordinating to ferrihy-drite and the uncomplexed/unprotonated As–O bond, respectively.The presence of protonated As–O–H bond could not be ruled out,since there appeared to be a weak feature at$700cmÀ1.It was interesting to note that all sorption samples from pH3to8had very similar sorption density(i.e.As/Fe$0.25,see Table1),but the surface arse-nate species varied from poorly crystalline ferric arsenate to bidentate–binuclear surface complexes as pH increased. This was indicative that the nature of surface arsenate spe-cies strongly depended on the pH of the sorption media.The spectra of pH3and4sorption samples showed strong sulfate bands between950and1250cmÀ1.Sulfate ions were apparently adsorbed onto the ferrihydrite during synthesis from sulfate medium and displaced by arsenate during adsorption as discussed elsewhere(Jia and Demopo-ulos,2005).At higher pH(i.e.5–8),the sulfate ions were substituted by arsenate ions as indicated by the absence of sulfate bands on the infrared spectra.For the adsorption systems of Fe/As=2,no sulfate band was observed for all pH samples(see Fig.1).Ferrihydrite synthesized from sulfate media was found to adsorb significantly more arsenate than that from nitrate media(Jia and Demopoulos,2005),which could not be ex-plained by the difference in BET surface areas.Therefore,it was of interest to compare the surface arsenate species of the two types of arsenate–ferrihydrite sorption samples. Fig.4shows the effect of pH on the infrared spectra of arsenate adsorbed on nitrate–ferrihydrite.Apparently the spectra are similar to those of arsenate adsorbed on sul-fate–ferrihydrite(Fig.1).Both are dominated by the strong As–O stretching vibration bands at700–950cmÀ1and strong O–H stretching vibration bands at3000–3500cmÀ1.In the As–O stretching vibration region(700–950cmÀ1),the infrared spectra of acidic sorption samples (pH3,4)exhibited a strong,well-resolved single band at similar position to that of poorly crystalline ferric arse-nate.This was indicative that a ferric arsenate surface precipitate formed when arsenate adsorbed on nitrate–fer-rihydrite at acidic pH,similar to As(V)adsorption onTable1Arsenic equilibrium concentration(mg/L)and sorption density(mol-As/mol-Fe)for the adsorption of arsenate on ferrihydrite synthesized from sulfate and nitrate medium at pH3–8and initial Fe/As molar ratio of2,4and8pH Fe/As=2Fe/As=4Fe/As=8Sulfate Nitrate Sulfate Nitrate Sulfate3198(0.49)318(0.46) 1.0(0.25) 1.9(0.25)<0.02(0.125) 4321(0.46)471(0.43) 1.9(0.25) 3.4(0.25)0.2(0.125) 5440(0.44)615(0.41) 3.6(0.25)14.8(0.25)0.2(0.125) 6498(0.43)788(0.38) 5.0(0.25)29.6(0.25)0.2(0.125) 7702(0.40)1070(0.32)15.8(0.25)100(0.24)0.6(0.125) 81178(0.30)1439(0.25)141(0.24)178(0.23) 2.1(0.125)The numbers in bracket are sorption densities,i.e.As/Fe molar ratio of the solids.1648Y.Jia et al./Geochimica et Cosmochimica Acta71(2007)1643–1654sulfate–ferrihydrite.As pH increased,the single As–O stretching vibration band split into two strong bands at $806–810cmÀ1and$878cmÀ1as observed for the sul-fate–ferrihydrite samples(see Fig.1)and were attributed to the As–O stretching vibration of bidentate binuclear complexed arsenate ions.The formation of a ferric arse-nate surface precipitate at acidic pH was also evidenced by the change of the$3190cmÀ1O–H band with pH.The spectrum of ferrihydrite displayed strong NO3Àbands at1250–1500cmÀ1.These bands disappeared after adsorption of arsenate indicating that nitrate ions previ-ously adsorbed during ferrihydrite synthesis were dis-placed by arsenate ions.3.2.Effect of coverage density on the nature of adsorbed arsenateThe effect of coverage density on the nature of adsorbed arsenate on ferrihydrite was evaluated.At acidic pH(i.e. pH3,4),the As–O stretching vibration region(700–950cmÀ1)was dominated by a strong well-resolved band (Fig.5).As the Fe/As molar ratio increased from2to8(i.e.the adsorption density As/Fe decreasing from0.49to0.125)for the pH3sorption systems(see Table1),the intensity of the As–O stretching vibration band decreased markedly.The As–O stretching vibration peak was located at similar position.The pH4arsenate–ferrihydrite sorptionThe nature of adsorbed arsenate on ferrihydrite1649samples with various arsenate sorption densities exhibited very similar infrared spectra to that of pH3samples.Poor-ly crystalline ferric arsenate was the major surface arsenate species.The nature of surface arsenate species was not sig-nificantly influenced by arsenate sorption density and equi-librium concentration.At pH6,the major As–O stretching vibration peak was located at$822cmÀ1for all the initial Fe/As molar ratios used,i.e.2,4and8.A visible shoulder was also emerging at $878cmÀ1on the infrared spectra.A bidentate complex of arsenate was becoming detectable in addition to the major surface species of ferric arsenate.When pH increased to8,all infrared spectra exhib-ited two bands at808and878cmÀ1irrespective of the initial Fe/As molar ratios indicating that bidentate com-plexes were the dominant surface arsenate species.The equilibrium concentration of arsenic ranged from0.028 to15.8mM for the pH8sorption systems and the sorp-tion density(As/Fe)ranged from0.125to0.31.Waych-unas et al.(1993)conducted EXAFS analysis on samples with sorption density of0.001–0.1and concluded that arsenate was sorbed via bidentate surface complexation. Goldberg and Johnston(2001)used FTIR to character-ize the arsenate–iron oxide sorption solids synthesized at pH5and9with equilibrium concentration of0.1–1.0mM and suspension density of4g/L($8g/L for the present work).The obtained results showed similar As–O band to the present study,i.e.two bands located at$817–824and$854–861cmÀ1.The infrared spectrum obtained by Roddick-Lanzilotta et al.(2002)for the sorption system pH2.6and arsenic equilibrium concen-tration of$0.5mM displayed a well-resolved band $825,which is similar to the single As–O band of the acidic samples in this work.Carlson et al.(2002)pro-posed the formation of ferric hydroxyarsenate in Fe/As=2.6–3.2sorption solids prepared from solutions of pH3.Therefore,by comparing the present study with other works,it is proposed that the pH plays the most impor-tant role in controlling the nature of the surface arsenate species sorbed on ferrihydrite,whereas other conditions (e.g.coverage density,suspension density etc.)play less important factors.The fate of sulfate in synthetic ferrihydrite before and after adsorption of arsenate can also be monitored on the infrared spectra.As indicated by the bands between950 and1250cmÀ1,more sulfate was incorporated into the fer-rihydrite at acidic pH(3,4)than neutral to alkaline pH (6,8)(Fig.5).After adsorption of arsenate at pH3,4 and Fe/As=4,8,there was still measurable amount of sul-fate remaining in the ferrihydrite,while at Fe/As=2,all sulfate has been desorbed.It was proposed that sulfate ions were adsorbed on goethite as both inner-sphere and outer-sphere surface complexes at acidic pH(Peak et al.,1999). Adsorption of arsenate on the ferrihydrite involved ligand exchange with previously adsorbed sulfate ions.With increasing pH,the adsorbed sulfate ions on ferrihydrite were more easily displaceable by arsenate.At pH6,sulfate bands were visible only on the Fe/As=8infrared spectrum while no sulfate was detectable by FTIR in the pH8sam-ples.This is in good agreement with a previous study that reported the quantitative analysis of the sorption solids (Jia and Demopoulos,2005).It was suggested that surface precipitation of phosphate and arsenate on goethite occurred almost simultaneously (Zhao and Stanforth,2001;Ler and Stanforth,2003).The process of surface precipitation may involve slow dissolution of ferrihydrite,ternary complexation of Fe3+ and subsequent precipitating of arsenate(Ler and Stan-forth,2003).The process was depicted schematically in Fig.6.It was not surprising that arsenate surface precipitate formed at such a high initial Fe/As molar ratio of8where arsenic equilibrium concentration is below the detection limit(<0.02mg/L)in this work.There possibly also existed tridentate complex structure that formed initially upon contacting of the arsenate ions with ferrihy-drite given the amorphous nature of the latter.The saturation state with respect to poorly crystalline ferric arsenate in acidic media was estimated in a previous study(Jia et al.,2006).When the p K sp(ferrihydrite)=39 (Langmuir,1997)and p K sp(ferric arsenate)=22.89 (Mahoney,2002)were taken in the calculation,the log IAPð½Fe3þ ½AsO43À was obviously lower than log K sp (ferric arsenate)for the pH3,Fe/As=4and Fe/As=8 sorption systems,indicating that the systems were undersat-urated with respect to poorly crystalline ferric arsenate.For。
天文词汇S——精选推荐
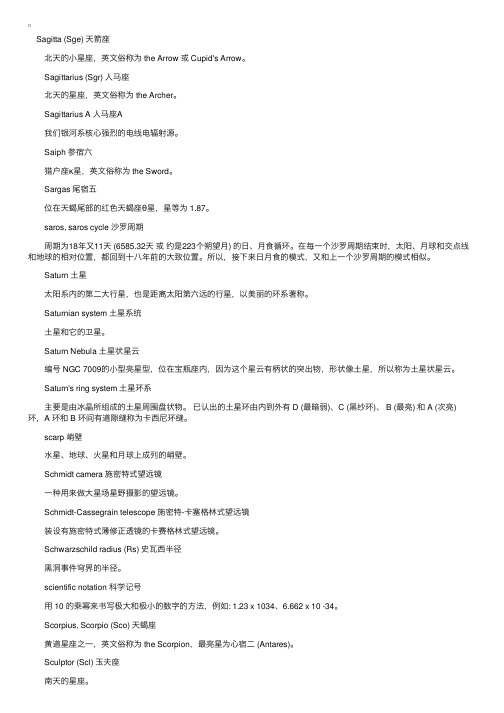
Sagitta (Sge) 天箭座 北天的⼩星座,英⽂俗称为 the Arrow 或 Cupid's Arrow。
Sagittarius (Sgr) ⼈马座 北天的星座,英⽂俗称为 the Archer。
Sagittarius A ⼈马座A 我们银河系核⼼强烈的电线电辐射源。
Saiph 参宿六 猎户座κ星,英⽂俗称为 the Sword。
Sargas 尾宿五 位在天蝎尾部的红⾊天蝎座θ星,星等为 1.87。
saros, saros cycle 沙罗周期 周期为18年⼜11天 (6585.32天或约是223个朔望⽉) 的⽇、⽉⾷循环。
在每⼀个沙罗周期结束时,太阳、⽉球和交点线和地球的相对位置,都回到⼗⼋年前的⼤致位置。
所以,接下来⽇⽉⾷的模式,⼜和上⼀个沙罗周期的模式相似。
Saturn ⼟星 太阳系内的第⼆⼤⾏星,也是距离太阳第六远的⾏星,以美丽的环系著称。
Saturnian system ⼟星系统 ⼟星和它的卫星。
Saturn Nebula ⼟星状星云 编号 NGC 7009的⼩型亮星型,位在宝瓶座内,因为这个星云有柄状的突出物,形状像⼟星,所以称为⼟星状星云。
Saturn's ring system ⼟星环系 主要是由冰晶所组成的⼟星周围盘状物。
已认出的⼟星环由内到外有 D (最暗弱)、C (⿊纱环)、 B (最亮) 和 A (次亮)环,A 环和 B 环间有道隙缝称为卡西尼环缝。
scarp 峭壁 ⽔星、地球、⽕星和⽉球上成列的峭壁。
Schmidt camera 施密特式望远镜 ⼀种⽤来做⼤星场星野摄影的望远镜。
Schmidt-Cassegrain telescope 施密特-卡塞格林式望远镜 装设有施密特式薄修正透镜的卡赛格林式望远镜。
Schwarzschild radius (Rs) 史⽡西半径 ⿊洞事件穹界的半径。
scientific notation 科学记号 ⽤ 10 的乘幂来书写极⼤和极⼩的数字的⽅法,例如: 1.23 x 1034、6.662 x 10 -34。
小学英语一年级Unit 10
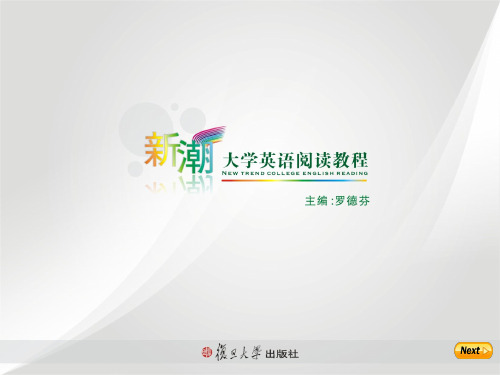
Spectroscopic analysis
a Spectroscope is an instrument
for forming and observing spectra. Spectroscopic analysis is an analysis of a spectrum to determine characteristics of its source, especially in the visible region of electromagnetic spectrum; for example, analysis of the optical spectrum of an incandescent body to determine its composition or motion.
―They will watch the flash through their lunar telescopes and get a spectroscopic analysis. That will tell them more than they know now about the atmosphere of our planet and the composition of its surface. They’ll be here in person within a few oppositions. And then —‖
外太空 应急供氧装臵 返回式卫星
通信卫星
遥感卫星 运载火箭 气象卫星 中国空间技术研究院 中国航天局
NASA (The National Aeronautics and Space Administration) 美国航空航天管理局
Earthmen Bearing Gifts
第四纪地质年代学-Cosmogenic_Dating
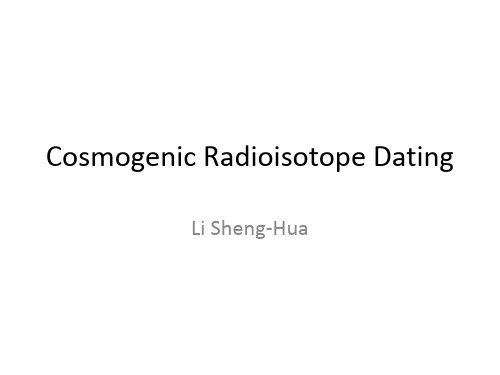
• Production rate P is constant through the age of interest, and known accurately
• No contamination from other materials • Known concentration (either N0 or
materials
materials
Charcoal,
Soil, fossils,
organic matters pollens
Quartz,
Rock surface
feldspar, olivine
Calcite
Rock surface
Quartz,
feldspar, olivine
Quartz,
l
Age equations
• Solving the equation: t = -1 ln(1- Nl )
l
P
• For a stable isotope, λ approaching to 0,
Surface exposure dating 10Be, 36Cl
t= N P
3He Dating
10k-2000ka 200-1000ka
Be-10, Cl-36 and Al-26 Dating
• Age affecting factors (Mainly production rate P)
– Variation of geomagnetic field strength – Erosion – Shielding – Chemical weathering – Geometry change – Elevation changes
基于医学影像PACS的数据管理系统
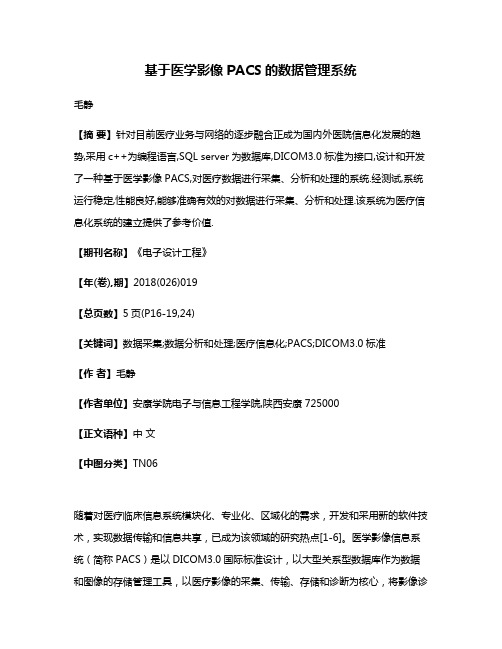
基于医学影像PACS的数据管理系统毛静【摘要】针对目前医疗业务与网络的逐步融合正成为国内外医院信息化发展的趋势,采用c++为编程语言,SQL server为数据库,DICOM3.0标准为接口,设计和开发了一种基于医学影像PACS,对医疗数据进行采集、分析和处理的系统.经测试,系统运行稳定,性能良好,能够准确有效的对数据进行采集、分析和处理.该系统为医疗信息化系统的建立提供了参考价值.【期刊名称】《电子设计工程》【年(卷),期】2018(026)019【总页数】5页(P16-19,24)【关键词】数据采集;数据分析和处理;医疗信息化;PACS;DICOM3.0标准【作者】毛静【作者单位】安康学院电子与信息工程学院,陕西安康725000【正文语种】中文【中图分类】TN06随着对医疗临床信息系统模块化、专业化、区域化的需求,开发和采用新的软件技术,实现数据传输和信息共享,已成为该领域的研究热点[1-6]。
医学影像信息系统(简称PACS)是以DICOM3.0国际标准设计,以大型关系型数据库作为数据和图像的存储管理工具,以医疗影像的采集、传输、存储和诊断为核心,将影像诊断查询、报告管理、集影像采集传输与存储管理、综合信息管理等综合应用于一体的综合系统[7-11]。
主要的功能是把医院影像科日常产生的各种医学影像数据通过DICOM标准接口,以数字化的方式海量保存起来,帮助医生快速使用图像资料,同时具备辅助诊断管理的功能[12-16]。
文中以C++为语言,SQLServer2008R2为数据库,DICOM3.0标准为接口,设计和实现了可以对医学影像数据进行采集和分析处理的系统。
1 系统设计1.1 SQL Server 2008 R2数据库本系统采用的SQLServer2008R2是基于SQLServer2008提供可靠高效的智能数据平台构建而成的数据库[17-19]。
SQLServer2008 R2提供了许多新改进,可帮助用户调整规模、提高IT效率并实现管理完善的自助BI。
- 1、下载文档前请自行甄别文档内容的完整性,平台不提供额外的编辑、内容补充、找答案等附加服务。
- 2、"仅部分预览"的文档,不可在线预览部分如存在完整性等问题,可反馈申请退款(可完整预览的文档不适用该条件!)。
- 3、如文档侵犯您的权益,请联系客服反馈,我们会尽快为您处理(人工客服工作时间:9:00-18:30)。
a rXiv:as tr o-ph/22178v18Fe b22REVISTA MEXICANA DE F ´ISICASpectroscopic Dating of Stellar Populations from Local Star Clusters to Distant Galaxies and the Age of the Universe ∗Gustavo Bruzual A.Centro de Investigaciones de Astronom´ıa,Apartado Postal 264,M´e rida 5101-A,Venezuela Recibido el 10de diciembre de 2001;aceptado el 1st February 2008I summarize in terms of evolutionary population synthesis models our current understanding of stellar populations in different environments,from star clusters in nearby galaxies to distant galaxies in clusters or seen through gravitational lenses.Constraints on the age of the stellar populations in clusters and galaxies derived from comparing predicted and observed spectral energy distributions are examined and contrasted with the maximum ages allowed by cosmological models at the corresponding redshift.Keywords:galaxies:general,galaxies:evolution,galaxies:stellar populations Presento un resumen del conocimiento actual de las propiedades de las poblaciones estelares presentes en diferentes ambientes,desde c´u mulos estelares en galaxias cercanas hasta galaxias lejanas en c´u mulos de galaxias o galaxias observadas gracias a lentes gravitacionales,en t´e rminos de modelos de s´ıntesis evolutiva de poblaciones estelares.Se examinan las restricciones que se pueden imponer en la edad de las poblaciones estelares en c´u mulos estelares y galaxias a partir de la comparaci´o n de espectros predichos y observados,y se contrastan con la m´a xima edad permitida por modelos cosmol´o gicos al correspondiente valor del corrimiento al rojo.Descriptores:galaxias:general,galaxias:evoluci´o n,galaxias:poblaciones estelares 1.Introduction Evolutionary population synthesis provides an important tool to study the stellar content of star clusters and galaxies as a func-tion of age.The theory of stellar evolution makes definitive predictions concerning the bolometric luminosity and effective temperature of a star of a given mass and chemical composition.As time proceeds,the function describing the radial depen-dence of the chemical composition of the material inside the star varies,resulting in changes in the bolometric luminosity and the effective temperature characterizing a star of a given initial mass.The evolutionary track for this stellar mass is the line joining these successive points in the bolometric luminosity-effective temperature plane,known as the Hertzsprung-Russell diagram (HRD).Complete sets of evolutionary tracks for stars of a wide mass range and various initial metal contents covering all significant stellar phases are available in the literature (Fagotto et al.1994a,b,c;Girardi et al.2000).Assuming an initial mass function (IMF,e.g.Salpeter 1955),we can compute the number of stars of a given mass born at time zero,and then follow the evolution of this population in the HRD using a specific set of evolutionary tracks.Knowledge of the spectral energy distribution (SED)at each position in the HRD visited by the stars during their evolution,allows us to compute the integrated SED for this initial-burst or simple stellar population (SSP)as a function of time.By means of a convolution integral (Bruzual &Charlot 1993)the evolution of the SED can be computed for an arbitrary star formation rate (SFR)and a chemical enrich-ment law.A detailed description of the evolutionary synthesis technique has been published by Bruzual (1999).A discussionof the most important results obtained from spectral evolutionary models is also presented in this paper,as well as in Bruzual (2001a,b).Several stellar spectral libraries are currently available.The Pickles (1998)atlas provides good coverage of the HRD for stars of solarmetallicity (Z ⊙)at medium spectral resolution.Recently,Le Borgne et al.(2002a)have compiled an equivalentatlas at 3˚A spectral resolution (1˚A sampling)which is complete for stars of solar metallicity,but includes a large number ofspectra of non-solar metallicity stars.On the theoretical side the compilation by Lejeune et al.(1997,1998)and Westera et al.(2002)provide libraries of model atmospheres for stars of various metallicities but at ≈20˚A spectral resolution.The last twolibraries are largely based on the Kurucz (1995)series of model stellar atmospheres.In a parallel paper (Bruzual 2002),I have discussed the advantages of using a high resolution stellar spectral library,such as the Le Borgne et al.(2002a)atlas,in a set of evolutionary population synthesis models (Bruzual &Charlot 2002,BC02hereafter).These models preserve the properties of models built at lower spectral resolution in what respects to integrated photometric properties of the stellar population,e.g.luminosity and color evolution,but achieve a much greater level of detail in reproducing the spectral features of the integrated population.This is particularly important when comparing model SEDs with observed spectra obtained with the high resolution spectrograph available in the Hubble Space Telescope (HST)and in the new generation of ground based high performance optical telescopes (Keck,VLT,Sloan).In this paper I show the results of2TITLEFig.1:Comparison of the observed SED of the young massive cluster W3in the galaxy NGC7252(kindly provided by F.Schweizer,heavyline)with a Z=Z⊙SSP at0.57Gyr computed for the Salpeter(1955)IMF using the Le Borgne et al.(2002a)stellar atlas(thin line).Themodel SED is the line extending to the extreme left and right in the plot.comparing the predictions of the BC02models with the observed SED of star clusters and galaxies seen at various cosmological epochs,as measured by their redshift(z).2.Spectroscopic Age of Stellar PopulationsIn this section I use the BC02models to derive the spectroscopic age that characterizes the observed SED of several sources at different distances from us.A model consists of a spectrum which evolves in time from age zero to20Gyr in221time steps. By minimizingχ2= [log Fλ(observed)−log Fλ(model)]2,we derive for each problem SED the(spectroscopic)age at which the model matches more closely the observed spectrum.2.1.Star ClustersFig1shows a comparison of a BC02model SED with the observed spectrum of the young massive(globular)cluster W3in the galaxy NGC7252,kindly provided by F.Schweizer.The model corresponds to a Z=Z⊙SSP at0.57Gyr computed for the Salpeter(1955)IMF using the Le Borgne et al.(2002a)stellar atlas.The spectra show a remarkable degree of agreement in the shape and intensity of the Hydrogen Balmer lines(Hβthrough H14),the Mgb feature,the Fe and other lines indicated in the plot.The intensity of the Balmer lines is an excellent indicator of the age of the stellar population,provided that the spectral resolution of both spectra match in this wavelength range.This is the case in Fig1.Models built with lower resolution libraries do not constrain the age of the cluster population so accurately.2.2.Nearby GalaxiesIn Fig2I show a VLT spectrum of an elliptical galaxy at z=0.142(kindly provided by J.-F.Le Borgne)in thefield of the cluster of galaxies AC114together with a BC02model that produces the best match to the observed SED at an age of12Gyr. The model is the same one shown in Fig1,but seen11.43Gyr later in its passive evolution.Many of the spectral features seen in the problem galaxy SED are real since they are also present in the stellar spectra used to build the model.2.3.Intermediate Distance GalaxiesStockton(2001)has identified galaxies dominated by old stellar populations at moderately high redshifts(z≈1.5).Presum-ably,these galaxies formed most of their stars early in the history of the universe and have evolved passively later on.The dataRev.Mex.F´ıs.AUTHORS 336003800400042004400460000.511.546004800500052005400560000.511.55600580060006200640066000.511.5Galaxy: ac114.1.3.17.0001, z = 0.14183, elliptical:PROBLEM GALAXY :MODEL GALAXY (12 Gyr)Fig.2:VLT spectrum of an elliptical galaxy at z =0.142gravitation-ally lensed in the field of the cluster of galaxies AC114(kindly pro-vided by J.-F.Le Borgne,heavy line)compared with the same modelof Fig 1but seen at 12Gyr (thin line).Fig.3:Broad band photometry in the galaxy rest frame for LBDS 53W091and six galaxies from Stockton’s sample compared to the model of Fig 1at the age indicated in each panel (thick line).The 1.4Gyr SED is repeated in all the frames (thin line)to guide the eye.points in the different frames of Fig 3show the broad band photometry in the galaxy rest frame for LBDS 53W091(Spinrad et al.1997),and six galaxies from Stockton’s sample.The colors or broad band fluxes of LBDS 53W091are well reproduced by the same solar metallicity,Salpeter IMF,BC02model SSP introduced above at an age of 1.4Gyr (top frame on the left hand side in Fig 3;see also Bruzual &Magris 1997).Two of the galaxies observed by Stockton have the colors of this population at 2Gyr,whereas the remaining 4galaxies seem older,≈4Gyr .The 1.4Gyr SED is repeated in all the frames as a guide to the eye in establishing the differences between the SEDs.Despite the photometric errors and the intrinsic uncertainties in the population synthesis technique,it seems safe to conclude that even though these 7galaxies are at very similar redshifts,their stellar populations differ in age by a factor of two.This may give us an indication on how precisely tuned star formation was at the time of formation of these galaxies.2.4.Distant Galaxies.A Passive Evolution SequenceFig 4shows in a concise way a comparison of the predicted passive evolution of the SED of a SSP at 6different epochs and observations (either broad band fluxes and/or SEDs)of various galaxies covering a wide range in redshift space.The age of the model that reproduces the observations is indicated in each panel.At one age extreme,object S2in the top panel of Fig 4,a young (6Myr)reddened starburst at z =1.87seen through a gravitational lens (Le Borgne et al.2002b),provides an example of a very young population at a cosmological epoch at which (elliptical?)galaxies dominated by old populations were already in place.Object H5(Pell´o et al.1999),shown in the second panel of Fig 4,at z =4.05is a quite distant galaxy also seen through a gravitational lens and is characterized by a stellar population with age close to 60Myr.Source 3by Cowie et al.(2001)at z =2.6is another lensed galaxy seen at an age close to an order of magnitude older than object H5.M32and an average nearby elliptical galaxy SED,shown in the two bottom panels in Fig 4,characterize present day local counterparts of the spectral energy distribution of old stellar populations.LBDS 53W091(Fig 3),reproduced in the 4th panel of Fig 4,provides a link between the younger and older than 1Gyr stellar populations.Fig 4thus shows a plausible evolutionary sequence of elliptical galaxy spectra.Rev.Mex.F´ıs.4TITLESpectral Evolution2000400060008000Fig.4:Plausible evolutionary sequence of elliptical galaxy spectra.Comparison of the predicted passive evolution of the SED of a SSPat6different epochs and observations(either broad bandfluxes and/orSEDs)of various galaxies covering a wide range in redshift space.Theage of the model that reproduces the observations is indicated in eachpanel.z (redshift)Age of spectroscopically dated galaxies and star clusters024651015Fig.5:Age of the universe as a function of redshift for two differentcosmological models:H0=70km s−1Mpc−1,ΩM=1,ΩΛ=0,t U(0)=9.33Gyr(bottom solid line),and H0=70km s−1Mpc−1,ΩM=0.28,ΩΛ=0.72,t U(0)=13.75Gyr(top solidline).The data points represent the ages determined spectroscopicallyfor the galaxies in Fig4and globular star clusters taken from the litera-ture.The open dots represent the galaxies in Stockton’s sample shownin Fig3.The vertical segments at z=0and z=1.55represent arange in possible ages and not an error bar.3.Spectroscopically Dated Galaxies and the Age of the UniverseFig5shows the age of the universe as a function of redshift for two different cosmological models.The data points represent the ages determined spectroscopically for the different galaxies shown in Figs3and4,as well as globular star clusters taken from the literature.It is clear that the age of local E galaxies and globular clusters,and possibly LBDS53W091and two of Stockton’s galaxies,rule out the H0=70km s−1Mpc−1,ΩM=1,ΩΛ=0universe with a maximum age t U(0)=9.33 Gyr at z=0(bottom solid line).On the other hand,the age of these systems and of all galaxies shown in Fig5,is below the age(z)line for the standard model,H0=70km s−1Mpc−1,ΩM=0.28,ΩΛ=0.72,t U(0)=13.75Gyr(top solid line).A selection effect is clear in Fig5,the sources that have been observed,except for the galaxies at z≈1.5,represent the youngest, and possibly the brightest galaxies at a given z,and may not be representative of the galaxy population as a whole in that shell of z-space.Equally young stellar populations seem to be present at all z’s,at least from z≥2to z≤4.4.DiscussionI have shown how evolutionary population synthesis models can be used to assign a spectroscopic age to stellar populations whose SED or multi-broad-band photometry is available.The error in the age determination depends on the quality of the observational data and on the uncertainties in the population synthesis models.In general,the relative error in the spectroscopic age increases with decreasing galaxy age.Despite this caveat,it is possible to establish a plausible sequence along which the spectra of galaxies may evolve when passive evolution dominates the evolution of simple stellar populations,at a rate that is consistent with that expected in the most accepted cosmological model describing our universe,H0=70km s−1Mpc−1,ΩM=0.28,ΩΛ=0.72,t U(0)=13.75Gyr.Rev.Mex.F´ıs.AUTHORS5 References1.Bica,E.,Alloin,D.,Bonatto,C.,Pastoriza,M.G.,Jablonka,P.,Schmidt,A.,&Schmitt,H.R.1996,in A Data Base forGalaxy Evolution Modeling,eds.C.Leitherer et al.,PASP,108,9962.Bruzual A.,G.1999,in Proceedings of the XI Canary Islands Winter School of Astrophysics on Galaxies at High Redshift,eds.I.P´e rez-Fournon and F.S´a nchez,Cambridge Contemporary Astrophysics,(in press)3.———.2001a,Astrophys.&Space Science277(Suppl.),2214.———.2001b,in Proceedings of the IAU Symposium No.207“Extragalactic Star Clusters”,eds.D.Geisler and E.Grebel,Astr.Soc.Pac.Conference Series,(in press)5.———.2002,Rev.Mex.Astron.Astrophys.Conf.Series,(in press)6.Bruzual A.,G.&Charlot,S.1993,ApJ,405,5387.———.2002,ApJ,in preparation(BC02)8.Bruzual A.,G.&Magris,G.1997,in Proceedings of the STScI May Symposium“The Hubble Deep Field”,eds.M.Livio,S.M.Fall&P.Madau,p.99.Cowie,L.L.,Barger,A.J.,Bautz,M.W.,Capak,P.,Crawford,C.S.,Fabian,A.C.,Hu,E.M.,Iwamuro,F.,Kneib,J.-P.,Maihara,T.,&Motohara,K.2001,ApJ,551,L910.Fagotto,F.,Bressan,A.,Bertelli,G.,&Chiosi,C.1994a,A&AS,100,64711.———.1994b,A&AS,104,36512.———.1994c,A&AS,105,2913.Girardi,L.,Bressan,A.,Bertelli,G.,&Chiosi,C.2000,A&AS,141,37114.Kurucz,R.1995,private communication15.Le Borgne,J.-F.,et al.2002a,A&A,(in preparation)16.Le Borgne,J.-F.,Schaerer,D.,Bruzual A.,G.,Pell´o,R.,Lemoine-Busserole,M.,Campusano,L.E.,Kneib,J.-P.,&Smail,I.,2002b,A&A,(in preparation)17.Lejeune,T.,Cuisinier,F.,&Buser,R.1997a,A&AS,125,22918.———.1998,A&AS,130,6519.Pell´o,R.,Kneib,J.P.,Le Borgne,J.F.,B´e zecourt,J.,Ebbels,T.M.,Tijera,I.,Bruzual A.,G.,Miralles,J.M.,Smail,I.,Soucail,G.,&Bridges,T.J.1999,A&A,346,35920.Pickles,A.J.1998,PASP,110,86321.Rieke,M.1997,private communication22.Salpeter,E.E.1955,ApJ,121,16123.Spinrad,H.,Day,H.,Stern,D.,Dunlop,J.,Peacock,J.,Jim´e nez,R.,&Windhorst,R.,1997,ApJ,484,58124.Stockton,J.2001,in Astrophysical Ages and Time Scales,eds.T.von Hippel,N.Manset,and C.Simpson,Astr.Soc.Pac.Conference Series,V ol.245,p.51725.Westera,P.,Lejeune,T.,Buser,R.,Cuisinier,F.,&Bruzual A.,G.2002,A&A,in pressRev.Mex.F´ıs.。