Microstructure and Compressive Properties of NbTiVTaAlx
Microstructure and properties of pure Ti processed by ECAP and cold extrusion
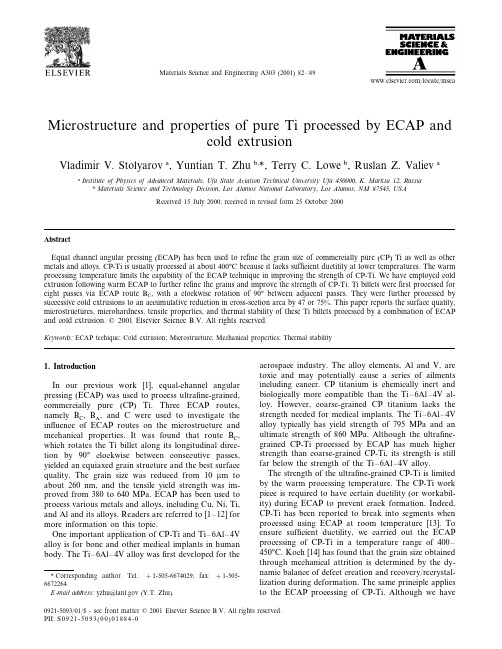
Materials Science and Engineering A303(2001)82–89Microstructure and properties of pure Ti processed by ECAP andcold extrusionVladimir V.Stolyarov a ,Yuntian T.Zhu b,*,Terry C.Lowe b ,Ruslan Z.Valiev aaInstitute of Physics of Ad 6anced Materials ,Ufa State A 6iation Technical Uni 6ersity Ufa 450000,K .Marksa 12,RussiabMaterials Science and Technology Di 6ision ,Los Alamos National Laboratory ,Los Alamos ,NM 87545,USAReceived 15July 2000;received in revised form 25October 2000AbstractEqual channel angular pressing (ECAP)has been used to refine the grain size of commercially pure (CP)Ti as well as other metals and alloys.CP-Ti is usually processed at about 400°C because it lacks sufficient ductility at lower temperatures.The warm processing temperature limits the capability of the ECAP technique in improving the strength of CP-Ti.We have employed cold extrusion following warm ECAP to further refine the grains and improve the strength of CP-Ti.Ti billets were first processed for eight passes via ECAP route B C ,with a clockwise rotation of 90°between adjacent passes.They were further processed by successive cold extrusions to an accumulative reduction in cross-section area by 47or 75%.This paper reports the surface quality,microstructures,microhardness,tensile properties,and thermal stability of these Ti billets processed by a combination of ECAP and cold extrusion.©2001Elsevier Science B.V.All rights reserved.Keywords :ECAP techique;Cold extrusion;Microstructure;Mechanical properties;Thermal stability /locate /msea1.IntroductionIn our previous work [1],equal-channel angular pressing (ECAP)was used to process ultrafine-grained,commercially pure (CP)Ti.Three ECAP routes,namely B C ,B A ,and C were used to investigate the influence of ECAP routes on the microstructure and mechanical properties.It was found that route B C ,which rotates the Ti billet along its longitudinal direc-tion by 90°clockwise between consecutive passes,yielded an equiaxed grain structure and the best surface quality.The grain size was reduced from 10m m to about 260nm,and the tensile yield strength was im-proved from 380to 640MPa.ECAP has been used to process various metals and alloys,including Cu,Ni,Ti,and Al and its alloys.Readers are referred to [1–12]for more information on this topic.One important application of CP-Ti and Ti–6Al–4V alloy is for bone and other medical implants in human body.The Ti–6Al–4V alloy was first developed for theaerospace industry.The alloy elements,Al and V,are toxic and may potentially cause a series of ailments including cancer.CP titanium is chemically inert and biologically more compatible than the Ti–6Al–4V al-loy.However,coarse-grained CP titanium lacks the strength needed for medical implants.The Ti–6Al–4V alloy typically has yield strength of 795MPa and an ultimate strength of 860MPa.Although the ultrafine-grained CP-Ti processed by ECAP has much higher strength than coarse-grained CP-Ti,its strength is still far below the strength of the Ti–6Al–4V alloy.The strength of the ultrafine-grained CP-Ti is limited by the warm processing temperature.The CP-Ti work piece is required to have certain ductility (or workabil-ity)during ECAP to prevent crack formation.Indeed,CP-Ti has been reported to break into segments when processed using ECAP at room temperature [13].To ensure sufficient ductility,we carried out the ECAP processing of CP-Ti in a temperature range of 400–450°C.Koch [14]has found that the grain size obtained through mechanical attrition is determined by the dy-namic balance of defect creation and recovery /recrystal-lization during deformation.The same principle applies to the ECAP processing of CP-Ti.Although we have*Corresponding author.Tel.:+1-505-6674029;fax:+1-505-6672264.E -mail address :yzhu@ (Y.T.Zhu).0921-5093/01/$-see front matter ©2001Elsevier Science B.V.All rights reserved.PII:S 0921-5093(00)01884-0V.V.Stolyaro6et al./Materials Science and Engineering A303(2001)82–8983not observed dynamic recrystallization in CP-Ti during the ECAP,we did observe dynamic recovery.Recovery is a thermally activated process,so higher processing temperatures will exponentially increase the rate of dynamic recovery.This results in larger grains and a lower dislocation density,and consequently lower strength.Another factor that affects the microstructure and workability is pressing speed[13,15].Increasing the strain rate during the ECAP may result in higher dislocation density andfiner grain size,since the recov-ery process is time-dependent.However,higher pressing rate will reduce the workability of CP-Ti,as observed by Semiatin et al.[13].The advantage of ECAP is its capability of maintain-ing the net dimensions of the work piece so that the process can be repeated to obtain the desired strain and grain size.Its disadvantage is the requirement of work piece to have high ductility,making it impossible to process CP-Ti at room temperature.As a comparison, most other high-strain processing methods,such as extrusion,rolling,and drawing,requires less ductility of the work piece and can process CP-Ti at room temper-ature.Consequently,these techniques may be able to introduce more defects such as dislocations into the work piece and improve its strength.However,these techniques do not preserve the dimensions of the work piece.One or more dimensions of the work piece are continuously reduced,which not only limits the obtain-able strain,but also eventually reduces the work piece to afinal geometry of foil/plate or wire,limiting their structural application[4,15].In addition,these tech-niques are not effective in refining grain size[16]. Therefore,it is unfeasible to use these techniques to process CP-Ti into high strength,while maintaining its useful dimensions for many structural applications.In our previous work[17],we have demonstrated that high-pressure torsion(HPT)at room temperature can further refine grain size and improve strength of CP-Ti processed by ECAP.However,HPT can only process small disc-shaped samples that are unsuitable for structural applications.In this investigation,we have combined the advantages of both ECAP and cold extrusion to further improve the strength of CP-Ti, while maintaining the work piece in structurally useful dimensions.CP-Ti rods werefirst processed by warm ECAP(route B C)to reduce the grain size,and then extruded at room temperature.The strength of CP-Ti processed by these steps was comparable to that of Ti–6Al–4V alloy.The resulting microstructures were thermally stable up to300°C.This paper reports the processing,microstructure,mechanical properties and thermal stability of ultrafine-grained CP-Ti processed by ECAP-cold extrusion(ECAP-CE).2.Experimental proceduresCommercially pure Ti with an average grain size of 10m m and containing impurities including0.12wt.% O,0.01wt.%H,0.04wt.%N,0.07wt.%C,and0.18 wt.%Fe was used as the starting material.The dimen-sions of the starting billets were26mm in diameter and 120mm in length.ECAP route B C,which rotates the work piece90°clockwise along its longitudinal axis between adjacent passes,was used to process the Ti billets.This route was chosen because it yields the best surface quality and equiaxed grains[1].The die channel angle was90°.The entrance channel had a diameter of 26mm and the exit channel has a diameter of25mm, which is slightly smaller than the entrance channel to allow easy reinsertion of the billet into the entrance channel in the following pass(see Fig.1).Molybdenum disulfide was used as a lubricant.Both Ti billet and die were preheated to450°C before the start of the ECAP process.All billets were processed for eight passes,with the starting temperature at450°C.The temperature dropped with each pass and reached400°C after the8th pass.The Ti billets processed by ECAP were machined to a dimension of22mm in diameter and80mm long to remove the surface defects and to create a smooth surfacefinish.A layer of natrium echoborium was then applied to the billet for lubrication during the subse-quent cold extrusion.The coated billets were extruded through a series of dies,progressively reducing the diameter from22to20to18to16mm(total reduction in cross-section area=47%)to14.3to12.6to11mm (total reduction in cross-section area=75%).The coat-ing was reapplied before each pass.All samples for transmission electron microscopy (TEM),microhardness and mechanical testing were cutFig.1.The ECAP die has a90°channel angle.The diameters of entrance and exit channels are26and25mm,respectively,to allow easy reinsertion of the billet into the entrance channel in the following pass.V .V .Stolyaro 6et al ./Materials Science and Engineering A 303(2001)82–8984Fig.2.CP-Ti billets processed by ECAP and cold extrusion have high surface quality.away from the ends of Ti billets and near the center in the transverse direction.To observe the grain shape and microstructural an-isotropy,transmission electron microscopy (TEM)sam-ples were cut from both the transverse and longitudinal-section.These samples were prepared by jet electropolishing.Bright and dark field TEM images were taken using a JEM-100B microscope.An acceler-ating voltage of 100KV was used.Electron diffraction patterns were taken from an area of 2m m 2.The average grain size was measured from the dark field image by number-averaging the diameters of more than 60grains.The microhardness was measured in both transverse and longitudinal sections by the Vicker’s method under a load of 100g for 10s.Ten measurements were made for each sample and their average was taken as the microhardness of the sample.Tensile tests were performed at room temperature using a universal testing machine IR-5047-50.Cylindri-cal samples [1]with a gauge section of 5mm in diameter and 25mm in length were machined from the Ti billets.A displacement rate of 1mm min −1.was used for all tests.Yield strength,ultimate strength,elongation to failure,and reduction in area at the necking cross-section was measured.To study the thermal stability,Ti billets processed by ECAP followed by cold extrusion (75%reduction in cross-section area)were annealed at 200–500°C for half an hour.Dog-bone shaped flat samples with a gage dimension of 2.8×0.3×10mm were cut from the longitudinal sections.The samples were cut by electro-spark cutting and electro-polished.Tensile testing of these samples were performed using a PV-3012machine at a displacement rate of 1mm min −1.3.Results3.1.Surface qualityShown in Fig.2are three CP-Ti billets processed by cold extrusion following initial ECAP.Two billets have a smooth surface without surface defects,while the third one has a crack near one end.These results indicate that it is feasible to extrude CP-Ti at room temperature following warm ECAP processing,al-though surface cracking could cause problems in some billets.3.2.MicrostructuresFig.3shows the TEM micrographs and selected area diffraction (SAD)pattern of Ti billet processed by ECAP route B C for eight passes.Fig.3a was taken from the transverse section of the as-processed billet while Fig.3b was from the longitudinal section.TheFig. 3.TEM micrographs and SAD pattern from (a)transverse section and (b)longitudinal section of a Ti billet processed by ECAP routes B C for eight passes.The initial diameter of the billet is 26mm.V.V.Stolyaro6et al./Materials Science and Engineering A303(2001)82–8985 SAD pattern,which was taken from the transversesection,indicates the existence of a large fraction ofhigh-angle grain boundaries.Clustered diffraction spotssuggests the existence of low-angle grain boundaries.Such a mixture of low-angle and high-angle grainboundaries is typical of ECAP-processed metals andalloys[2–4,17–21].It can be seen from Fig.3b that thegrains are somewhat elongated in the longitudinal di-rection.This is in contrast with our previous report[1]that ECAP route B C yielded equiaxed grains.Thisdifference could have been caused by the size effect.The Ti billets processed in the previous report[1]were20mm in diameter,while the Ti billets in the currentinvestigation are26mm in diameter.This observationmay suggest potential complication in scaling ECAPprocessing to increasingly large diameter billets.It isnot clear if such a size effect occurs in other materials.Figs.4and5show the microstructures of Ti billetsprocessed by ECAP followed by cold extrusion at roomtemperature for total reductions in cross-section areaby47and75%,respectively.No intermediate annealingFig. 5.TEM micrographs and SAD pattern from(a)transversesection and(b)longitudinal section of a Ti billet processed by ECAProutes B C for eight passes,followed by cold extrusion for a reductionin cross-section area of75%(diameter reduction from22to11mm).Fig. 4.TEM micrographs and SAD pattern from(a)transverse section and(b)longitudinal section of a Ti billet processed by ECAP routes B C for eight passes,followed by cold extrusion for a reduction in cross-section area of47%(diameter reduction from22to16mm).was performed between cold extrusion passes.Fig.4a, which is from the transverse section,shows a grain size similar to that shown in Fig.3a,indicating that the47% reduction in cross-section area from cold extrusion did not significantly change the grain size.However,Fig.4a shows higher dislocation density and less sharp grain boundaries than Fig.3a.Fig.4b shows elongated grains,higher dislocation density and less sharp grain boundaries than Fig.3b.Further cold extrusion to a reduction in cross-section area of75%resulted in irregular grain shapes in the transverse section(Fig.5a)and elongated grain shape in the longitudinal section(Fig.5b).In addition,the grain size as measured from the transverse section(Fig. 5a)is much smaller than observed after47%reduction in cross-section area(Fig.4a).Fig.5indicates the formation offibrous grains.Numerous diffraction spots indicate that most grain boundaries are high angle, while the elongation of the diffraction spots indicates the existence of internal elastic strain.V.V.Stolyaro6et al./Materials Science and Engineering A303(2001)82–89 86The Ti billets cold-extruded to75%reduction in cross-section area were annealed at280°C for1h to check the microstructural stability.Fig.6shows the annealed microstructure from the longitudinal cross paring Fig.6with Fig.5b reveals that the fibrous nature of the grains was mostly maintained,and the grain boundaries are clearly defined.Although not clear in Fig.6,the decrease in dislocation density should be lower in Fig.6than in Fig.5due to recovery processes during the annealing.3.3.Mechanical properties3.3.1.MicrohardnessTable1lists the microhardness of the Ti billets after various processing steps.Each microhardness value is an average of at least10measurements.The scatter of the measured values is about5%or less.It is obvious that cold deformation after the ECAP further increases the microhardness.In addition,the microhardness in-creases with increasing reduction in cross-section area from the cold extrusion.Another observation is that the microhardness measured from the longitudinal sec-tion is higher than from the transverse section for all deformation states.To investigate the uniformity of microhardness,Ti rods with a dimension of40mm in diameter and200 mm in length were processed by ECAP route B C for eight passes.Microhardness was measured along the length near the center axis of the Ti billet in a longitu-dinal section as well as from the center to the surface on a cross-section.It is found that the microhardness is uniform along the length and shows a1%increase only at2mm away from the surface.Therefore,the micro-hardness is uniform.3.3.2.Tensile propertiesTensile tests were conducted on cylindrical samples with gauge dimensions of5mm in diameter and25mm in length.The testing results are listed in Table 2. Readers are referred to[1]for more details on the specimen geometry.The longitudinal axis of the sample coincides with that of the billets.After cold extrusion to 47%reduction in cross-section area,the yield and ulti-mate strength were increased to910and930MPa, respectively,which are higher than those of Ti–6Al–4V alloy.Further cold extrusion to a reduction in cross-section area of75%yielded even higher yield and ultimate strengths.3.4.Thermal stabilityTo study the thermal stability,Ti billets,processed by ECAP route B C for eight passes followed by cold extrusion for a reduction in cross-section area of75, were annealed at200–500°C for half an hour.Shown in Fig.7are tensile stress strain curves obtained from smallflat samples,which have a gage dimension of 2.8×0.3×10mm.The sample longitudinal axis coin-cides with the longitudinal axis of Ti billet.The as-pro-Fig.6.TEM micrographs and SAD pattern from the longitudinal section of a Ti billet processed by ECAP routes B C for eight passes, followed by cold extrusion for a reduction in cross-section area of 75%,and then annealed at280°C for1h.Table1The microhardness measured from both transverse and longitudinal sections of Ti billets after various processing steps aDeformation state Microhardness(MPa)inTransverse Longitudinalsectionsection2700ECAP b281029402880ECAP+Cold extrusion(47%c)33503230ECAP+Cold extrusion(75%)a The values in MPa were converted from Vickers measurements.b Route BC for eight passes.c Reduction in cross-section area from cold extrusion.Table2Yield strength(|0.2),ultimate strength(|u),elongation to failure(l), and reduction in area( )of Ti in various processing stateECAP route (%)|0.2(MPa)|u(MPa)l(%)Coarse-grained(grain2738069460size=10m m)1471061640ECAP a–ECAP+Cold55910930extrusion(47%b)8105097042 ECAP+Coldextrusion(75%)2510860795Ti–6Al–4V ca Route BC for eight passes.b Reduction in cross-section area from cold extrusion.c From ASTM F136–96.V .V .Stolyaro 6et al ./Materials Science and Engineering A 303(2001)82–8987Fig.7.Typical tensile stress-strain curves for CP-Ti processed by ECAP route B C for eight passes,followed by cold extrusion for a reduction in cross-section area of 75%,and then annealed at varying temperatures for 0.5h.Small flat samples with a gage dimension of 2.8×0.3×10mm were used.The sample longitudinal axis coincides with the longitudinal axis of Ti billet.tively high processing temperature (400–450°C).For example,the yield strength of CP-Ti obtained by ECAP alone is only 640MPa.A low processing temperature is desired to introduce finer grains and higher dislocation density into the CP-Ti,and to consequently further improve strength.However,the ECAP process requires the work piece to have relatively high ductility,which rules out the processing of CP-Ti using ECAP at room temperature [13].This work has shown that a two-step process,warm ECAP followed by cold extrusion,can effectively fur-ther improve the strength of CP-Ti while maintaining a useful work-piece dimension for structural applications.The ECAP significantly refines the grain size without changing the dimension of the work piece,while cold extrusion further improves the strength.The two-step process combined two strengthening mechanisms to strengthen CP-Ti:(a)grain boundary strengthening dueFig.8.The thermal stability of strength and the recovery of ductility with increasing annealing temperature.The CP-Ti was processed by ECAP route B C for eight passes,followed by cold extrusion for a reduction in cross-section area by 47or 75%,and then annealed at varying temperatures for 0.5h.CE strain in the legend means reduction in cross-section area from cold extrusion.cessed sample shows a very high strength but low elongation to failure.Annealing at temperatures up to 300°C for half an hour improved the ductility without significantly decreasing the ultimate strength.The thermal stability of strength and the recovery of ductility with increasing annealing temperature are shown in Fig.8.The CP-Ti samples were processed by ECAP route B C for eight passes,cold extruded for a reduction in cross-section area by 47or 75%,and then annealed at varying temperatures for half an hour.As shown,the yield and ultimate strength are stable at annealing temperatures up to 300°C.It can also be seen that the higher yield and ultimate strength produced by higher reduction in cross-section area from cold extru-sion were well preserved at annealing temperatures up to 400°C.Higher reduction in cross-section area from cold extrusion resulted in lower ductility in the as-pro-cessed state and annealed states.4.DiscussionThis investigation shows that very high yield strength (970MPa)and ultimate strength (1050MPa)as well as considerable ductility (elongation to failure =8%)can be attained in bulk,ultrafine-grained CP-Ti material processed by a combination of warm ECAP and cold extrusion.Warm ECAP has limited capability in refining grains and improving the strength of CP-Ti,due to the rela-V.V.Stolyaro6et al./Materials Science and Engineering A303(2001)82–89 88to small grain size;and(b)dislocation strengthening from cold deformation.Moreover,thefine grains re-sulted from the ECAP helped to maintain a good ductility even after significant straining from cold extrusion.The thermal stability of the ultrafine-grained CP-Ti produced by the two-step process is an important is-sue for structural applications.As shown in Fig.8, the strength of the CP-Ti processed by ECAP+cold extrusion is stable at temperatures up to300°C, which is remarkable considering that it was cold ex-truded at room temperature.Thermal annealing after the cold extrusion at temperatures below300°C is beneficial since it improves the ductility without sig-nificantly decreasing the strength.The thermal stabil-ity of mechanical properties of CP-Ti processed by ECAP+cold extrusion is not as high as those pro-cessed by ECAP only.In our previous work[1],the mechanical properties of CP-Ti processed by ECAP are stable at temperature up to400–450°C,which is the temperature range in which the CT-Ti was pro-cessed.This is because the microstructure is largely stable at temperatures below the processing tempera-ture.A salient feature of the microstructures of CP-Ti processed by ECAP and subsequent cold extrusion is that grains elongate in the longitudinal direction.The cold extrusion both increased dislocation density and elongated grains along the sample axis(Fig.5)as compared with a nearly equiaxed grain structure after ECAP(Fig.3).The grain elongation made grains ap-pearfibrous after a large reduction in cross-section (75%)from cold extrusion(Fig.5b).The observed anisotropy of grain shape is consistent with the mi-crohardness anisotropy in the transverse and longitu-dinal sections.Thefibrous nature of the microstructure is more clearly shown in Fig.6after the as-processed sample (75%reduction in cross-section)was annealed at 280°C for1h.The transverse diameter of thefibrous grains is estimated as about120nm.The annealing reduced the dislocation density through recovery,and the grain boundaries become more distinct.The lack of recrystallization and grain growth at280°C is con-sistent with the thermal stability of tensile strength, which is stable at temperature up to300°C.The high strength after cold extrusion suggests that the combination of ECAP and cold extrusion is a viable technique to produce high strength CP-Ti for structural applications.The same technique can be applied to other metals and alloys.The disadvantage of this technique is that the work piece needs to be well lubricated by coating before each cold extrusion pass and surface cracks may develop in some samples even with the aforementioned coating.Other cold de-formation techniques such as cold rolling are not so demanding in good lubrication,and may have pro-cessing advantages over cold extrusion.This is being investigated in our laboratories and will be reported in a future paper.5.SummariesA two step process combining ECAP and cold ex-trusion has been developed to process CP-Ti.The first step,ECAP,significantly refined the grain size and increased the yield and ultimate strength to640 and710MPa,respectively,without changing the sam-ple dimension.The second step,cold extrusion,fur-ther introduced higher dislocation density,and elongated the grains,which consequently increased the yield and ultimate strength to970and1050MPa, respectively.This two-step process makes use of two strengthening mechanisms to enhance the strength:(a)fine grain size;and(b)high dislocation density.The strength of the as-processed Ti is thermally stable at temperatures up to300°C.Annealing at temperatures below300°C resulted in improved ductility without significant decrease in strength.This study suggests that the ECAP+cold extrusion is a viable technique for producing ultrafine-grained materials with high strength for structural applications.References[1]V.V.Stolyarov,Y.T.Zhu,I.V.Alexandrov,T.C.Lowe,R.Z.Valiev,Influence of ECAP routes on the Microstructure and Properties of Pure Ti,Mater.Sci.Eng.A.(in press).[2]S.Ferrasse,V.M.Segal,K.T.Hartwig,R.E.Goforth,Metall.Mater.Trans.28A(1997)1047.[3]S.Ferrasse,V.M.Segal,K.T.Hartwig,R.E.Goforth,J.Mater.Res.12(1997)1253.[4]P.B.Prangnell,A.Gholinia,V.M.Markushev,in:T.C.Lowe,R.Z.Valiev(Eds.),Investigations and Applications of Severe Plastic Deformation,Kluwer Academia,Dordrecht,2000,pp.65–71.[5]Y.Iwahashi,Z.Horita,M.Nemoto,ngdon,ActaMater.46(1998)3317.[6]K.Oh-Ishi,Z.Horita,M.Furukawa,M.Nemoto,ng-don,Metall.Mater.Trans.29A(1998)2011.[7]K.Nakashima,Z.Horita,M.Nemoto,ngdon,ActaMater.46(1998)1589.[8]Y.Iwahashi,Z.Horita,M.Nemoto,ngdon,Metall.Mater.Trans.29A(1998)2503.[9]ngdon,M.Furukawa,Z.Horita,M.Nemoto,JOM50(June)(1998)41.[10]Y.Iwahashi,M.Furukawa,Z.Horita,M.Nemoto,ng-don,Metall.Mater.Trans.29A(1998)2245.[11]Y.Iwahashi,Z.Horita,M.Nemoto,ngdon,ActaMater.45(1997)4733.[12]V.M.Segal,Mater.Sci.Eng.A197(1995)157.[13]S.L.Semiatin,V.M.Segal,R.E.Goforth,N.D.Frey,D.P.Delo,Metall.Mater.Trans.30A(1999)1425.[14]C.C.Koch,NanoStructured Mater.2(1993)109.V.V.Stolyaro6et al./Materials Science and Engineering A303(2001)82–8989[15]F.J.Humphreys,P.B.Prangnell,J.R.Bowen,A.Gholinia,C.Harris,Phil.Trans.R.Soc.Lond.A357(1999)1663.[16]R.Z.Valiev,R.K.Islamgaliev,I.V.Alexandrov,Progress inMater.Sci.45(2000)103.[17]V.V.Stolyarov,Y.T.Zhu,T.C.Lowe,R.K.Islamgaliev,R.Z.Valiev,NanoStructured Mater.11(1999)947.[18]I.V.Alexandrov,Y.T.Zhu,T.C.Lowe,R.K.Islamgaliev,R.Z.Valiev,Metall.Mater.Trans.29A(1998)1047.[19]M.Furukawa,Y.Iwahashi,Z.Horita,M.Nemoto,N.K.Tsenev,R.Z.Valiev,ngdon,Acta Mater.45(1997) 4751.[20]M.Furukawa,Z.Horita,M.Nemoto,R.Z.Valiev,T.G.Langdon,Acta Mater.44(1996)4619.[21]J.R.Weertman and S.R.Agnew,A progress report for theperiod of Feb.1to28,1997.Submitted to Los Alamos Na-tional Lab.,Contract c7764Q0016-35..。
岩石细观结构及参数对宏观力学特性及破坏演化的影响_张国凯_李海波_夏祥_李俊如_
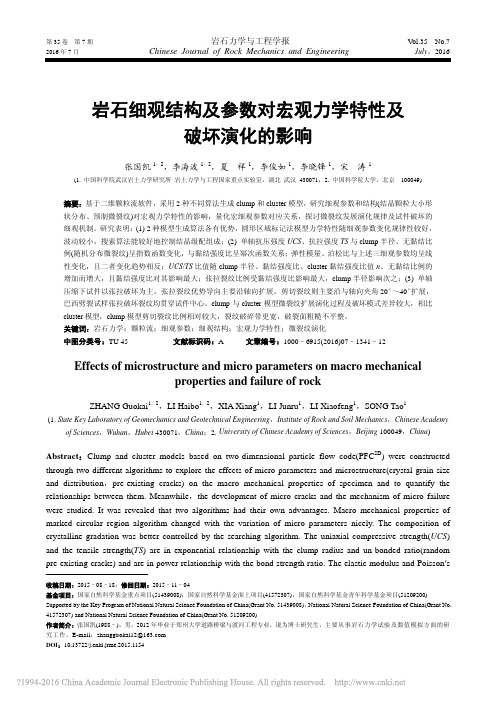
Effects of microstructure and micro parameters on macro mechanical properties and failure of rock
ZHANG Guokai1 2,LI Haibo1 2,XIA Xiang1,LI Junru1,LI Xiaofeng1,SONG Tao1
第 35 卷 第 7 期 2016 年 7 月
岩石力学与工程学报 Chinese Journal of Rock Mechanics and Engineering
Vol.35 No.7 July,2016
岩石细观结构及参数对宏观力学特性及 破坏演化的影响
难熔金属高熵合金的制备工艺和性能研究开题报告

毕业论文开题报告毕业论文开题报告题目:难熔金属高熵合金的制备工艺和性能研究学院:物理电气信息学院专业:物理学(师范)年级:2010级学号:12010245509姓名:刘永强指导教师:汪燕青邮箱:874322801@开题报告几千年来,合金的发展都是以一种金属元素为主的(一般都超过50%),随着添加各种不同的合金元素而产生不同的合金,以满足所需的性能要求,例如以铝为主的铝合金,以铁为主的钢铁材料,以铜为主的铜合金,以镍为主的高温合金,以钛为主的钛合金等等。
尽管如此,合金系的数量还是很有限的,目前人类已开发使用的合金系共有30余种。
一、难熔金属及高熵合金的定义、基本原理难容金属一般指熔点高于1650℃并有一定储量的金属(钨、钽、钼、铌、铪、铬、钒、锆和钛),也有将熔点高于锆熔点(1852℃)的金属称为难熔金属。
以这些金属为基体,添加其他元素形成的合金称为难熔金属合金。
制造耐1093℃(2000°F )以上高温的结构材料所使用的难熔金属主要是钨、钼、钽和铌。
在难熔金属合金中钼合金是最早用作结构材料的合金,Mo-0.5Ti-0.1Zr-0.02C 合金具有良好的高温强度和低温塑性,在工业上广泛应用。
铌合金的出现迟于钼合金,但发展很快,已有30余种牌号。
航天工业中使用的主要是中强合金和低强高塑性的铌合金。
高熵合金是1995年台湾学者叶均蔚提出的一个新的合金设计理念[1-3]。
与传统合金设计理念不同的是,高熵合金是由五个主要元素(简称为主元)构成的合金体系,且每个主元的原子百分比应介于5%到35%之间,而原子百分比小于5%的元素则称之为次要元素。
根据传统合金设计观念,合金中的组元数越多,形成金属间化合物的倾向也就越大。
然而脆性金属间化合物的出现会恶化合金的性能,如变脆等,同时金属间化合物的晶体结构复杂,也不利于材料的组织和成分分析。
因此传统合金都是以一种元素为主,添加少量次要元素改进其性质。
但是物极必返,叶均蔚指出,当主元数尽可能的增大后,所带来的高熵效应却可以化繁为简,令合金形成一个或多个简单的固溶相,不仅便于分析,而且具有巨大的应用价值。
Influence of micro-blasting on the microstructure and residual stresses of CVD κ-Al2O3 coatings
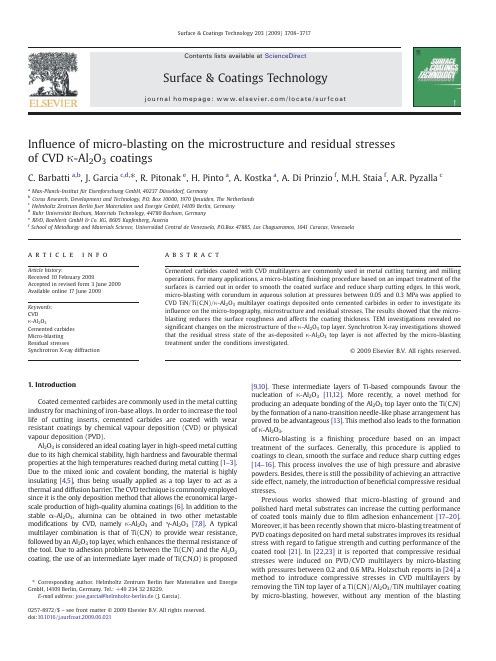
In fluence of micro-blasting on the microstructure and residual stresses of CVD κ-Al 2O 3coatingsC.Barbatti a ,b ,J.Garcia c ,d ,⁎,R.Pitonak e ,H.Pinto a ,A.Kostka a ,A.Di Prinzio f ,M.H.Staia f ,A.R.Pyzalla caMax-Planck-Institut für Eisenforschung GmbH,40237Düsseldorf,GermanybCorus Research,Development and Technology,P.O.Box 10000,1970IJmuiden,The Netherlands cHelmholtz Zentrum Berlin fuer Materialien und Energie GmbH,14109Berlin,Germany dRuhr Universität Bochum,Materials Technology,44780Bochum,Germany eR&D,Boehlerit GmbH &Co.KG,8605Kapfenberg,Austria fSchool of Metallurgy and Materials Science,Universidad Central de Venezuela,P.O.Box 47885,Los Chaguaramos,1041Caracas,Venezuelaa b s t r a c ta r t i c l e i n f o Article history:Received 10February 2009Accepted in revised form 3June 2009Available online 17June 2009Keywords:CVD κ-Al 2O 3Cemented carbides Micro-blasting Residual stressesSynchrotron X-ray diffractionCemented carbides coated with CVD multilayers are commonly used in metal cutting turning and milling operations.For many applications,a micro-blasting finishing procedure based on an impact treatment of the surfaces is carried out in order to smooth the coated surface and reduce sharp cutting edges.In this work,micro-blasting with corundum in aqueous solution at pressures between 0.05and 0.3MPa was applied to CVD TiN/Ti(C,N)/κ-Al 2O 3multilayer coatings deposited onto cemented carbides in order to investigate its in fluence on the micro-topography,microstructure and residual stresses.The results showed that the micro-blasting reduces the surface roughness and affects the coating thickness.TEM investigations revealed no signi ficant changes on the microstructure of the κ-Al 2O 3top layer.Synchrotron X-ray investigations showed that the residual stress state of the as-deposited κ-Al 2O 3top layer is not affected by the micro-blasting treatment under the conditions investigated.©2009Elsevier B.V.All rights reserved.1.IntroductionCoated cemented carbides are commonly used in the metal cutting industry for machining of iron-base alloys.In order to increase the tool life of cutting inserts,cemented carbides are coated with wear resistant coatings by chemical vapour deposition (CVD)or physical vapour deposition (PVD).Al 2O 3is considered an ideal coating layer in high-speed metal cutting due to its high chemical stability,high hardness and favourable thermal properties at the high temperatures reached during metal cutting [1–3].Due to the mixed ionic and covalent bonding,the material is highly insulating [4,5],thus being usually applied as a top layer to act as a thermal and diffusion barrier.The CVD technique is commonly employed since it is the only deposition method that allows the economical large-scale production of high-quality alumina coatings [6].In addition to the stable α-Al 2O 3,alumina can be obtained in two other metastable modi fications by CVD,namely κ-Al 2O 3and γ-Al 2O 3[7,8].A typical multilayer combination is that of Ti(C,N)to provide wear resistance,followed by an Al 2O 3top layer,which enhances the thermal resistance of the tool.Due to adhesion problems between the Ti(C,N)and the Al 2O 3coating,the use of an intermediate layer made of Ti(C,N,O)is proposed[9,10].These intermediate layers of Ti-based compounds favour the nucleation of κ-Al 2O 3[11,12].More recently,a novel method for producing an adequate bonding of the Al 2O 3top layer onto the Ti(C,N)by the formation of a nano-transition needle-like phase arrangement has proved to be advantageous [13].This method also leads to the formation of κ-Al 2O 3.Micro-blasting is a finishing procedure based on an impact treatment of the surfaces.Generally,this procedure is applied to coatings to clean,smooth the surface and reduce sharp cutting edges [14–16].This process involves the use of high pressure and abrasive powders.Besides,there is still the possibility of achieving an attractive side effect,namely,the introduction of bene ficial compressive residual stresses.Previous works showed that micro-blasting of ground and polished hard metal substrates can increase the cutting performance of coated tools mainly due to film adhesion enhancement [17–20].Moreover,it has been recently shown that micro-blasting treatment of PVD coatings deposited on hard metal substrates improves its residual stress with regard to fatigue strength and cutting performance of the coated tool [21].In [22,23]it is reported that compressive residual stresses were induced on PVD/CVD multilayers by micro-blasting with pressures between 0.2and 0.6MPa.Holzschuh reports in [24]a method to introduce compressive stresses in CVD multilayers by removing the TiN top layer of a Ti(C,N)/Al 2O 3/TiN multilayer coating by micro-blasting,however,without any mention of the blastingSurface &Coatings Technology 203(2009)3708–3717⁎Corresponding author.Helmholtz Zentrum Berlin fuer Materialien und Energie GmbH,14109Berlin,Germany.Tel.:+492343228229.E-mail address:jose.garcia@helmholtz-berlin.de (J.Garcia).0257-8972/$–see front matter ©2009Elsevier B.V.All rights reserved.doi:10.1016/j.surfcoat.2009.06.021Contents lists available at ScienceDirectSurface &Coatings Technologyj o u r n a l h om e p a g e :w w w.e l s ev i e r.c o m /l o c a t e /s u r fc o a tconditions employed.In[22–24]improvements of tool life of micro-blasted Al2O3coated cutting inserts in metal cutting are presented. The reason for the increment of the tool life is reported to be related to the introduction of compressive residual stresses on the Al2O3top layer.Although not mentioned in their publications,it is to be understood from the results presented that the authors worked with α-Al2O3coatings.Nevertheless,in spite of the many publications,no systematic study on the influence of micro-blasting pressure on Al2O3coating is found in the literature.In this work,we applied micro-blasting to CVD multilayer TiN/Ti (C,N)/κ-Al2O3coatings.The micro-blasting pressure range varies between0.05and0.3MPa.We aim at investigating systematically the influence of the blasting pressure on the micro-topography and the possibility of inducing compressive residual stresses on theκ-Al2O3 top layer.The microstructure and residual stress state of theκ-Al2O3top layer were investigated for the as-deposited and micro-blasted conditions by scanning electron microscopy,transmission electron microscopy,synchrotron as well as laboratory source X-ray diffraction and roughness measurements.2.Experimental2.1.Substrate and coatingsThe coatings were deposited onto cemented carbide substrates (90wt.%WC–10wt.%Co)with a size of14mm×14mm×5mm, produced by state-of-the-art powder metallurgy sintering techniques.A TiN/Ti(C,N)/κ-Al2O3multilayer was applied on the hard metal substrate by CVD in a standard hot-wall CVD reactor working at 1015°C and15kPa.The TiN and Ti(C,N)layers were deposited from the gas mixture TiCl4–CH4–N2–H2.The Al2O3layer was deposited on top of the Ti(C,N)layer from the gas mixture AlCl3–ClH2–CO2.Due to the deposition conditions employed,κ-Al2O3was produced as a top coating layer.2.2.Micro-blastingAfter deposition,the coatings were subjected to a micro-blasting treatment performed using an injector-type system in a commercial industrial blasting furnace.Standard industrial parameters were employed.The grit blasting material used was320-mesh(average diameter within~50µm)corundum shots in aqueous solution.The micro-blasting treatment was carried out for30s to ensure full coverage of the surfaces.The pressure was varied within the range from0.05MPa to0.3MPa in steps of0.05MPa.2.3.MicroscopyScanning electron microscopy(SEM)was performed on non-polished surfaces and fractured cross-sections.Specimens for transmission electron microscopy(TEM)analyses were prepared by using a gallium source JEOL JEM-9320focused ion beam(FIB)system operating at30kV.A JEOL2200F TEM/STEM operating at200kV and equipped with an EDX system was used for studying the microstructure of coatings in the as-deposited and micro-blasted state.2.4.RoughnessThe surface roughness(R a and R z)of as-deposited and micro-blasted coatings was determined from atomic force microscopy pictures.Four areas were measured in order to determine an average value.The measurements were carried out in a Nano-scratch tester equipped with an atomic force microscope(CSM Instruments).2.5.Phase and residual stress analysesIn order to assess the phase constitution within the as-deposited and micro-blasted coatings,X-ray diffraction(XRD)diffractograms were obtained using synchrotron radiation at the experimental station G3at DORIS III at HASYLAB at DESY,Hamburg.The radiation energy was 6.9keV(λCo=1.7904A˚´).The beam size was set as 4mm×1mm.The residual stresses within theκ-Al2O3coatings were determined on a laboratory four-circle diffractometer operating with Co–K a radiation and parallel-beam geometry using the sin2ψmethod[25]. Owing to the weak macroscopic texture of theκ-Al2O3coating, unrestricted lattice spacing measurements could be performed for24 sample directions defined by the inclination angleψbetween0°and 89.8°at the azimuthsφ=0°and180°.Considering the stress state in the coatings to be biaxial and of rotational symmetry,the fundamental relationship of X-ray stress analysis can be solved to provide the depth-dependence of the in-plane residual stressesσ//[26]:σ==τeffðÞ=eψτeffðÞ12s21ÀÁð1Þwhereɛψis the measured lattice strain,σ//is the in-plane residual stress,τeff is the information depth,and1/2s2and s1are the phase-specific diffraction elastic constants(DEC).The concept of effective information depthτeff is usually applied in diffraction studies of thinfilms to define the depth from which the diffraction information comes,since the penetration depthτof the radiation into a certain material may be much larger than thefilm thickness.According to its definitionτeff can be tuned within thefilm thickness by changing theψ-angle[27]:τeff=R DzÁe−zτd zRe−zτd z=τ−De−Dτ1−e−Dτð2Þwhereτis the average penetration depth for an infinitely thick sample,which is a function of theψ-tilt,and D is the thickness of theκ-Al2O3layer.In this approach the maximum achievable information depth approaches the limit of half layer thickness(D/2) as the ratio D/τin Eq.(2)decreases towards zero.The lattice strains were measured for the{135}diffraction line of κ-Al2O3.This reflection was chosen based on a compromise between the ease for separating this line from neighboring ones,its intensity and the highest possible2θ-angle.The DEC were estimated using the mechanical values of Young's modulus E=361.7GPa and Poisson's ratioν=0.24forκ-Al2O3at room temperature available in[5].The integral breadth of the selected reflection was also determined as a function of the micro-blasting conditions.This quantity is related to the density of crystalline defects(dislocations,stacking faults)and small size of crystallites(i.e.domains over which diffraction is coherent)[28].The integral breadth for each condition represents an average on the results obtained at differentψ-angles byfitting the {135}diffraction lines using pseudo-Voigt functions.3.Results3.1.Microstructure of the as-deposited coatingsThe as-deposited layer system is shown in Fig.1.The CVD TiN/ TiCN/κ-Al2O3layers show good adhesion to the cemented carbides, hence no pores,brittle phase formation nor spallation between the substrate and the coating are observed.The layer thickness is homogeneous across the coating,the thickness of the TiN is of about 0.2µm,for the intermediate Ti(C,N)layers is about3µm and the thickness of theκ-Al2O3is about4µm±0.05.3709C.Barbatti et al./Surface&Coatings Technology203(2009)3708–3717The alumina layer is composed of columnar grains that grow directly from the nucleation surface (here,the intermediate Ti(C,N)layer).The length of the columnar grains is mostly equivalent to the thickness of the alumina layer.A change in the morphology towards the surface can be observed,with the formation of hexagonal-shaped grains (Fig.1b and c).Some cracks (mainly transgranular)can also be observed.They are typical cracks as a consequence of the different thermal expansion coef ficients between the substrate and the coating and cannot really be avoided in commercial CVD cutting inserts.Several isolated large grains with average diameter larger than 5µmformed as a single crystal or a polycrystalline structure in a random distribution throughout the surface (Fig.2).Their number increases towards the edge of the inserts.Crack initiation seems to be associated with these outgrowths.The average surface roughness R a measured was 0.110±0.008(Table 1).The penetration depth of the X-rays in alumina is about 18µm.Hence,we have full penetration of the radiation into the coating system.The X-ray diffraction (XRD)patterns for the as-deposited sample are shown in Fig.3.The contribution of the substrate is also registered,indicated by the peaks associated with WC re flections.The XRD measurements also reveal that the intermediate layer is composed of Ti (C 0.3N 0.7).The intensity ratio between peaks shows no marked texture in the layers.Although the observation of the κ-→α-Al 2O 3phase transformation already during the deposition process is generally reported in literature [29],there is no evidence for the occurrence of such transformation,since the XRD diffractograms only revealed re flections associated with κ-Al 2O 3.This indicates that the super ficial cracks observed in the as-deposited coatings arise upon cooling due to the differences in thermal coef ficient between the coating and the substrate,and not originating from volume contraction that occurs in phase transformation.Fig.4shows a STEM micrograph of the as-deposited coating.The darkest areas at the bottom correspond to the cemented carbide substrate (WC grains are seen in black).The TiN layer with a thickness of 0.5µm lies adjacent to the substrate.Onto the TiN layer,the Ti(C,N)layer shows a changing grain morphology (Fig.4),making it appear as two distinct structures.The first sub-layer consists of columnar grains with a width of about 0.1µm.The following sub-layer has signi ficantly smaller grains with a needle-like morphology and appears randomly oriented.The uppermost layer consists of κ-Al 2O 3(in lighter contrast)and shows grains grown along the CVD deposition direction perpendicular to the substrate.The columnar microstructure istypicalFig.2.SEM image (BSE mode)of κ-Al 2O 3surface topography (top view)after coating.Isolated large Al 2O 3grains (with average size of 5µm)form in a random distribution throughout the surface.Crack initiation seems to be associated with these large grains (see arrows around the large Al 2O 3grain).Table 1Values of R a ,R z and thickness for the κ-Al 2O 3top layer as a function of micro-blasting pressure.Micro-blasting pressure [MPa]R a roughness [µm]R z roughness [µm]Thickness [µm]00.110±0.0080.690±0.04 4.20±0.050.050.096±0.0050.540±0.04 4.20±0.050.10.079±0.0060.480±0.04 4.00±0.050.150.038±0.0040.190±0.03 3.40±0.050.20.045±0.0030.250±0.03 3.00±0.050.250.074±0.0040.440±0.03 2.50±0.050.30.084±0.0040.540±0.042.50±0.05Fig.1.SEM images of (a)fracture cross-section of the coated cemented carbide,(b)top view of the surface topography of as-deposited CVD κ-Al 2O 3coating and (c)higher magni fication (top view)of the surface topography showing hexagonal-shaped grains.3710 C.Barbatti et al./Surface &Coatings Technology 203(2009)3708–3717for coatings deposited at low gas pressures and low temperatures compared to the melting point temperature [30].Besides the STEM mode,conventional Bragg diffraction contrast TEM was applied to investigate the evidence of defects within the κ-Al 2O 3top layer.A TEM micrograph in bright field (BF)mode is shown in Fig.5a.No dislocations but a high concentration of planar defects (twin-related faults)were observed (Fig.5a).All obtained electron diffraction patterns(e.g.Fig.5b)only reveal the presence of the κ-Al 2O 3phase.No evidence of phase transformation was obtained.The two possible variations of alumina (i.e.the metastable κ-Al 2O 3and the stable polymorph α-Al 2O 3)have strikingly different symmetry,which could have been easily distinguished if the latter were present.EDX analysis reveals almost no chemical intermixing,which suggests good separation of the layers.3.2.Microstructure of micro-blasted coatingsMicrographs of surface morphology and fracture cross-section of the samples micro-blasted at pressures in the range 0.05–0.15MPa are presented in Fig.6.Lower micro-blasting pressures,i.e.0.05and 0.1MPa cause no signi ficant changes in grain morphology,although a decrease in the average roughness is measured.At blasting pressures as low as 0.05MPa few isolated areas already appear deformed.As from 0.15MPa,signi ficant loss of coating material begins to occur and the number of cracks increases.Crack propagation typically occurs in a transgranular mode,but seems to be con fined to the near-surface zone of the upper coating.Micro-blasting at 0.2MPa causes a flattening of the grains and flaking of the coating as a consequence of the strong abrasive effect due to micro-blasting (Fig.7).Higher micro-blasting pressures (0.25and 0.3MPa)lead to strong changes in the surfacemicro-topographyFig.3.X-ray diffractogram of the as-deposited κ-Al 2O 3/Ti(C,N)coatings taken at ψ=0°with synchrotron radiation energy of 6.9keV (λCo =1.7904A˚´).The arrow indicates the position of the κ-Al 2O 3(135)re flection used for the residual stressevaluation.Fig. 4.(a)STEM micrograph showing the cross-section microstructure of the as-deposited coating.(b)Higher magni fication of the top κ-Al 2O 3layer.Fig.5.TEM micrograph in bright field (BF)mode showing planar defects within the as-deposited κ-Al 2O 3layer.(b)Selected area diffraction pattern (SADP)corresponding to κ-Al 2O 3.3711C.Barbatti et al./Surface &Coatings Technology 203(2009)3708–3717and an increase in the number and length of the cracks.The microstructure of the Ti(C,N)intermediate layer does not seem affected by the impact of the alumina shots.STEM micrographs of the cross-section microstructure of coatings micro-blasted at 0.1MPa and 0.3MPa are shown in Fig.8.By comparing the microstructure of the micro-blasted samples at different conditions,the only difference corresponds to the signi ficant reduced thickness of the alumina layer micro-blasted at 0.3MPa.The in fluence of micro-blasting pressure on the thickness of the κ-Al 2O 3layer is shown in Table 1.On the other hand,the results clearly show that the micro-blasting does not affect the adherence of the layer.Moreover,the Ti(C,N)is not affected by the mechanical treatment,the reduction in layer thickness being con fined to the top alumina layer.The TEM micrograph in BF mode (Fig.8c)shows the typical planar defects also observed within the alumina layer of the micro-blasted coatings (here we only show the example of the sample micro-blasted at 0.3MPa).The concentration of the twin-related faults observed in samples in all conditions seems to be identical,which directly suggests that the micro-blasting process has no in fluence on the defect density observed in the top κ-Al 2O 3layers.Besides,not a single dislocation was observed in the analyzed micro-blasted top κ-Al 2O 3layers.Micro-blasting leads to a decrease in the roughness of the alumina coating,showing a minimum at blasting pressures between 0.15and 0.2MPa (Table 1).Increasing the blasting pressure above 0.2MPa increases the roughness due to deterioration of the coating layer.ThisFig.6.SEM micrographs showing the changes on the κ-Al 2O 3thickness (fracture cross-sections)and surface topography (top view images)as a function of micro-blasting pressure:(a)and (b)at 0.05MPa;(c)and (d)at 0.1MPa;(e)and (f)at 0.15MPa.3712 C.Barbatti et al./Surface &Coatings Technology 203(2009)3708–3717is related to the extensive loss of the top layer material that occurs with increasing pressures.The removal of top coating material causes an increase in the line intensity of the WC re flections,since more contribution from the substrate is being registered (Fig.9).No changes in the intensity ratio between peaks with increasing micro-blasting pressures are observed.3.3.Residual stress analysesThe sin 2ψcurves of the κ-Al 2O 3layers in the as-deposited and micro-blasted (at 0.1and 0.3MPa)conditions are presented in Fig.10.The obtained d –sin 2ψdistributions are shown to be linear with positive slopes up to sin 2ψ~0.92in all conditions,indicating the presence of average residual stresses of tensile nature within the κ-Al 2O 3layer,which still predominate in the micro-blasted state.On top of the coatings (at large ψangles,thus,at small penetration depths),however,the d ψhkl–sin 2ψdistributions tend to curve downwards,suggesting the presence of near-surface gradients of the in-plane residual stresses in the as-deposited as well as in the micro-blasted conditions.ψ-splittingin the d ψhkl–sin 2ψplots does not occur,indicating the absence of shear stress components in all cases investigated.The in-plane residual stresses averaged over the entire κ-Al 2O 3layer are 355±41MPa,349±45MPa,and 392±39MPa in the as-deposited,0.1and 0.3MPa conditions,respectively (Table 2).Based on the sin 2ψdistributions (Fig.10),the in-depth distribution of the residual stresses in the κ-Al 2O 3coatings was determined using Eq.(1).The stress-depth pro files for the as-deposited and micro-blasted coatings are similar as presented in Fig.11.In the as-depositedstate,Fig.7.SEM micrographs showing the changes on the κ-Al 2O 3thickness (fracture cross-sections)and surface topography (top view images)as a function of micro-blasting pressure:(a)and (b)at 0.2MPa;(c)and (d)at 0.25MPa;(e)and (f)at 0.3MPa.3713C.Barbatti et al./Surface &Coatings Technology 203(2009)3708–3717the residual stress in the ‘bulk ’of the layer was found to be σ//(τ≈1.5µm)=699MPa,whereas the obtained value at the outer surface decreases down to σ//(τ≈0)=−32MPa.This indicates thatthe stresses become gradually less tensile towards the free surface,eventually turning into low compression at the surface.A similar behaviour is observed for the specimen micro-blasted at 0.1MPa:the tensile residual stress within the coating is slightly lower σ//(τ≈1.5µm)=603MPa,whereas at the surface low compressive residual stresses σ//(τ≈0)=−42MPa are also obtained.For the specimen micro-blasted at 0.3MPa,the stress values are σ//(τ≈1.5µm)=546MPa and σ//(τ≈0)=153MPa within the coating and close to the surface,respectively (Table 2).The analysis of the average integral breadth of the {135}diffraction line of κ-Al 2O 3suggests that the density of lattice imperfections does not signi ficantly increase with increasing micro-blasting pressures.This information corroborates the TEM investigations,which reveal low dislocation densities,even with increasing micro-blasting pressures.4.Discussion4.1.Effect of micro-blasting on microstructure and roughnessOur results indicate that the micro-blasting procedure only affects the κ-Al 2O 3top coating layer.SEM and TEM images show that there are no disturbances in the integrity of the intermediate Ti(C,N)layer.Strong deformation of the coatings appears as a flattening effect on the near-surface zone,mainly at pressures higher than 0.1MPa.ThisFig.8.STEM micrographs showing the cross-section microstructure of coatings micro-blasted at (a)0.1MPa and (b)0.3MPa.(c)TEM micrograph (BF)showing planar defects within the alumina layer of the coating micro-blasted at 0.3MPa.Fig.9.X-ray diffractograms of micro-blasted coatings as a function of micro-blasting pressure showing no change in the intensity ratio between peaks with increasing micro-blasting pressures.3714 C.Barbatti et al./Surface &Coatings Technology 203(2009)3708–3717flattening is produced on the one hand by the overlapping of the several localized deformations caused by the impact of the alumina shots on the surface.On the other hand,the impacts also remove material from the alumina coating,which also results in a smoothing of the protruding irregularities on the surface (Table 1).The lower roughness in the contact zone of the coating decreases the mechanical contact between asperities of the tribological pair tool-workpiece.This leads to a lower friction coef ficient and thus,a contribution for extended tool life of the inserts.4.2.Origin of stresses and defects4.2.1.Stress development upon CVD depositionCVD coatings are known to evolve relevant thermal mismatch residual stresses upon cooling from the high deposition temperatures [31].The strain and stress state in the layers change due to thermal strain introduced by differences in the coef ficients of thermal expansion between the individual layers and the substrate.The maximum thermal stresses induced by cooling (without taking stress relieving processes into account)can be estimated by using the Dietzel equation [32]:σc =E c αc −αs ðÞΔT f g =1−v c ðÞ+1−v s ðÞ=E s ½ d c =d s f gð3Þwhere αis the linear coef ficient of thermal expansion,νis the Poisson ratio,E is the Young's modulus,d is the thickness,c stands for coating and s for substrate.The thermal expansion coef ficient(TEC)Fig.10.The sin 2ψdistributions of the κ-Al 2O 3(135)re flection for φ=0°of the coatings (a)as-deposited,(b)micro-blasted at 0.1MPa and (c)micro-blasted at 0.3MPa.Table 2Values of internal stresses in the κ-Al 2O 3top layer as a function of micro-blasting pressure.Micro-blasting pressure [MPa]Average in-plane residual stresses [MPa]Stress value near the outer surface of κ-Al 2O 3[MPa]Stress value in the “bulk ”of κ-Al 2O 3[MPa]0355±41σ//(τ≈0)=−32σ//(τ≈1.5µm)=6990.1349±45σ//(τ≈0)=−42σ//(τ≈1.5µm)=6030.3392±39σ//(τ≈0)=153σ//(τ≈1.5µm)=546Fig.11.Depth pro file of the in-plane residual stresses obtained from the evaluation of the sin 2ψdistributions shown in Fig.11for the coatings (a)as-deposited,(b)micro-blasted at 0.1MPa and (c)micro-blasted at 0.3MPa.3715C.Barbatti et al./Surface &Coatings Technology 203(2009)3708–3717ofκ-Al2O3and Ti(C,N)usually lies within8.0–9.0×10−6K−1[33], whereas in the case of WC–Co cemented carbides it is in the range within 4.0–7.0×10−6K−1depending on the composition[34]. Hence,tensile stresses will necessarily develop in theκ-Al2O3coating sinceακ-Al2O3Nαs.Our results clearly show that average tensile stresses develop within theκ-Al2O3layer,indicating that thermal strains represent the major source of residual stresses in the present case.The low compressive stress values towards the surface could be attributed to the relaxation of the tensile residual stresses existent in the‘bulk’of the layer,since the interaction between the substrate and the coatings tends to decrease when approaching the free surface. Besides,the crack propagation,which seems to be confined to the zone closest to the surface,might also play a role in the relaxation mechanism.4.2.2.Stresses and defects induced by micro-blastingThe residual stresses are generally classified into three types: macro-stresses,micro-stresses,and root mean square(r.m.s.)stresses [25].Macro-stresses often develop during sample manufacturing, extending homogeneously over macroscopic distances,i.e.several grains.In contrast,micro-stresses arise over microscopic volumes, typically grain sizes,due to the mismatches in thermal and mechanical properties of the individual phases or grain orientations. Both cause diffraction line shifts since they are related to average lattice strains of grains with specific orientations.As discussed in the Section4.2.1,they are of thermal origin in the present case,and are not affected by the applied micro-blasting treatment.The third type of stresses(r.m.s.stresses),which is inhomogeneous within subgrains or crystallites due to the presence of lattice imperfections and vary on the atomic scale,does not induce line shifts but only diffraction line broadening,since any disturbance in the regularity of the crystal lattice is reflected in lattice parameter fluctuations.Typical causes for diffraction line broadening are therefore lattice distortions that arise from crystal defects(e.g.dislocations and planar faults),changes in the diffraction coherence length(domain size)and domain-size distribution[28].Based on evidences provided by both TEM and XRD investigations, we conclude that theκ-Al2O3top layer does not undergo plastic deformation during micro-blasting.In particular,TEM imaging indicates practically the absence of dislocations,even at a micro-blasting pressure as high as0.3MPa,which promotes extensive loss of theκ-Al2O3layer and strong changes in layer micro-topography.Planar defects in the form of twin-related faults are the only type of crystalline defects observed and already predominate in the as-deposited state,the density of which is not affected by the micro-blasting process.The crystal structure of the metastableκ-Al2O3is well established. It has an orthorhombic crystal structure(space group Pna21)with lattice parameters a=4.844Å,b=8.330Å,c=8.955Åformed from a pseudo-closed-packed stacking ABAC of oxygen atoms with Al in octahedral and tetrahedral environments in a3:1ratio[35,36]. Supposedly,due to the rather complex crystalline structure,distortion of the lattice occurs differently according to the crystallographic direction leading to twinning as the predominant deformation mechanism inκ-Al2O3phase in contrast toα-Al2O3,in which dislocation is the type of recurrent defects observed.5.ConclusionsMicro-blasting at pressures ranging from0.05up to0.3MPa was applied to CVDκ-Al2O3/Ti(C,N)/TiN multilayer coatings in order to investigate the potentiality of this procedure to control the surface micro-topography and modify the residual stress state of the coating. Our investigations reveal that:1.Theκ-Al2O3growth occurs in columnar direction,perpendicular tothe substrate.Noκ→αphase transformation of the Al2O3isinduced by micro-blasting according to both synchrotron X-ray diffraction and TEM studies.2.The effects of the micro-blasting treatment on the TiN/Ti(C,N)/κ-Al2O3multilayer under the conditions investigated are con-fined to theκ-Al2O3top layer,as revealed by the TEM and STEM investigations.3.Increasing the micro-blasting pressure leads to the formation andpropagation of cracks.SEM and TEM investigations show that crack propagation is confined to the zone closest to the surface.4.Increasing micro-blasting pressure leads to a reduction in roughnessfrom R a:0.110±0.008/R z:0.690±0.04in the as-deposited coatings up to R a:0.038±0.004/R z:0.190±0.03in coatings micro-blasted at0.15MPa,which is mainly associated with the loss of coating material.Blasting pressures above0.2MPa lead to coating degradation.5.TEM investigations showed high density of planar defects in theκ-Al2O3in the form of twin-related faults(Figs.5and8).TEM and XRD investigations reveal that theκ-Al2O3top layer does not undergo plastic deformation during micro-blasting.6.The residual stresses are of tensile nature and thermally induced andare not affected by the applied micro-blasting treatment.The average stress values over theκ-Al2O3layer are355±41MPa,349±45MPa, and392±39MPa in the as-deposited,0.1MPa and0.3MPa conditions.These tensile stresses ease towards the free surface.It can be in general concluded that,under the conditions investigated,the more remarkable effect of the micro-blasting treat-ment on theκ-Al2O3top layer was a reduction of the surface roughness for blasting pressures up to0.15MPa and a strong coating degradation for blasting pressures above0.2MPa.Neither the morphology nor the residual stress state of theκ-Al2O3top layer was affected by the micro-blasting.This is a main difference in the response to the treatment compared with the reported values forα-Al2O3[22,24,26],pointing out that the generation of compressive internal stresses by micro-blasting depends strongly on the crystalline structure of the Al2O3phase.This observation may be supported by previous investigations indicating a higher degree of plasticity ofα-Al2O3[37]compared toκ-Al2O3.On the other hand,the different physical characteristics of the metastable CVD κ-Al2O3compared to the CVDα-Al2O3,such as a smaller grain size,a lower pore density and an epitaxial growth,may also have an influence on the internal stress conditions after micro-blasting treatment[35]. AcknowledgementsThe authors(J.Garcia and H.Pinto)thank thefinancial support of the DFG project444Bra-113/25/0-1to carry out part of this work.Anna di Prinzio thanks ADEMAT Network,Alfa-project Nr.II-0240-B1-AT-RT-CT forfinancial support.Dr.A.Rothkirch(Hasylab),Dr.C.Juricic and Mr.P. Brito(MPIE)are kindly acknowledged for their support during the synchrotron X-ray measurements.Thanks to Mr.U.Föckeler(Ruhr Universität Bochum)for atom force microscopy measurements. References[1]F.Bunshah,Handbook of Hard Coatings,Noyes Publications/William AndrewPublishing,LLC,Norwich,New York,USA,2001.[2]P.K.Mehrothra,D.T.Quinto,High Temp.High Press.18(1980)199.[3]B.M.Kramer,N.P.Suh,J.Eng.Ind.102(1980)303.[4]R.H.French,J.Am.Ceram.Soc.73(1990)477.[5]B.Holm,R.Ahuja,Y.Yourdshhyan,B.Johansson,B.I.Lundqvist,Phys.Rev.,B59(1999)12777.[6]S.Ruppi,Int.J.Refract.Met.Hard Mater.23(2005)306.[7]S.Ruppi,rsson,Thin Solid Films388(2001)50.[8]S.Ruppi,rsson,A.Flink,Thin Solid Films516(2008)5959.[9]H.Holzschuh,Patent US6,436,519B2,20.08.2002.[10]H.Halvarsson,S.Vuorinen,Surf.Coat.Technol.56(1993)165.[11]M.Halvarsson,H.Nordén,S.Vuorinen,Surf.Coat.Technol.68–69(1994)266.[12]M.Halvarsson,J.E.Trancik,S.Ruppi,Int.J.Refract.Met.Hard Mater.24(2006)32.[13]R.Pitonak,J.Garcia,R.Weissenbacher,K.Udier,Patent EP1948842,30.07.2008.[14]D.M.Kennedy,J.Vahey,D.Hanney,Mater.Des.26(2005)203.[15]J.Qu,A.J.Shih,R.O.Scattergood,J.Luo,J.Mater.Process.Technol.166(2005)440.3716 C.Barbatti et al./Surface&Coatings Technology203(2009)3708–3717。
红柱石基刚玉-莫来石复相陶瓷的显微结构及性能

2 0范围为10-80。,扫描速度为4。/min,步宽为0.02*。
2结果分析
2.1 XRD分析 图1是不同温度下烧成后所得样品的Ⅺ①图谱。由图1
可见,在1450℃时,物相有刚玉、莫来石、红柱石和硅线石相; 随着温度达到1480℃,物相变为刚玉、莫来石和硅线石相,说 明红柱石相已完全转化,一部分发生了莫来石化过程,另一部 分转化成硅线石,硅线石的存在使得试样存在莫来石潜能,高 温作用时可以补偿试样产生的体积收缩;当温度达到15200c 时,物相已完全转变成剐玉和莫来石,表明低温生成的硅线石 相在高温下也转化为莫来石。 2.2 SEM分析
2010年第5期
n)pg
p0蛋茸(96)
宵dl乏一霉萤有鼍嗣土=I
Sintcring temperamre(oC) (4)
Sintering temperamre(。C) o,)
图3烧成温度对体密、气孔率的影响(a)和对抗折强度、耐压强度的影响(b) Fig.3 Effects of sintenng temperature on bulk density and open porosity Ca)and flexural and compressive strength(b)
能的影响.陶瓷学报.2006,27(1) ll程本军,郭兴忠,杨辉.徽粉及烧成温度对刚玉莫来石材料结构的
2010年第5期
中国陶瓷工业
9
影响.浙江大学学报,2006,40(8) 12程本军.郭兴忠,杨辉.刚玉莫来石复相陶瓷力学性能的影响因素
分析.材料科学与工程学报,2005,23(5)
13程本军,郭兴忠,杨辉.刚玉莫来石复相陶瓷热震及蠕变性能的影 响因素分析.硅酸盐通报,2005,(3)
用.矿物岩石地球化学通报.1999,18(4) 8郭敬娜,林先桥,乇渝斌.红柱石的粒度对奠来石一刚玉材料性能的
Microstructures and properties of Al0.3CoCrFeNiMnx high-entropy

Microstructures and properties of Al 0.3CoCrFeNiMn x high-entropy alloysSze-Kwan Wong,Tao-Tsung Shun *,Chieh-Hsiang Chang,Che-Fu LeeDepartment of Materials Science and Engineering,Feng Chia University,Taichung,407,Taiwanh i g h l i g h t sAll of the alloys exhibit an FCC polycrystalline structure.Addition of Mn to the alloy increases the lattice parameter,hardness,yield stress,and ultimate tensile strength. Solid-solution strengthening and work hardening are two mechanisms behind the strengthening. The addition of Mn to the alloy leads to a slight deterioration of corrosion resistance.a r t i c l e i n f oArticle history:Received 27March 2017Received in revised form 11July 2017Accepted 31July 2017Available online xxxKeywords:High-entropy alloy MicrostructureMechanical propertiesElectrochemical corrosion propertiesa b s t r a c tThe purpose of this study is to investigate the effects of Mn addition on the microstructure,mechanical properties,and electrochemical corrosion properties of Al 0.3CoCrFeNiMn x (x ¼0,0.1,and 0.3)high-entropy alloys.All alloys are simple face-centered cubic solid solutions.After addition of Mn to the Al 0.3CoCrFeNi alloy,the lattice parameter and hardness increase from 3.591Åto 3.611Åand from HV141to HV156,respectively.Meanwhile,the yield stress and ultimate tensile strength increase from 119MP to 158MPa and from 295MPa to 371MPa,respectively.The increase in yield stress is due to solid solution strengthening,and the increase in ultimate tensile strength results from the increase in work hardening due to the reduction of stacking fault energy.Polarization curves of the alloys show that the corrosion resistance slightly decreases after additions of Mn.©2017Elsevier B.V.All rights reserved.1.IntroductionDevelopments in traditional alloy systems have focused on one or two principal elements with atomic percentages of more than 50%.High-entropy alloys,a concept proposed by Yeh et al.,in 2004,comprise a new domain in alloy design [1].These alloys typically consist of five to thirteen principal metallic elements,the concen-tration of which range 5e 35at.%.The alloys ’properties can also be modi fied by adding minor elements such as interstitial C or B [2e 5].AlCoCrFeNi-based alloys with a combination of Cu,Ti,Mo,or V have been extensively studied because of the easy formation of the simple body-centered cubic (BCC)or face-centered cubic (FCC)matrix.These alloys show several attractive properties such as easy formation of nanostructure,high strength,good thermal stability,and high malleability [6e 10].Mn,one of the cheap metallic elements,is abundant in the earth ’s crust and is easy to utilize.It is widely used as an alloying element [11].For instance,it can be added to steel to enhance the abilities to resist impact and wear.It can be used to substitute Ni in stainless steel in order to reduce cost.It can also increase the hardening ability of aluminum alloys.In studies on Mn-containing high-entropy alloys,adding Mn to BCC AlCrFeCoNiCu alloy resulted in the formation of a Cr-rich phase,dramatically decreasing the alloy ’s compressive strength and ductility [12].However,the tensile strength of FCC CoCrFeMnNi alloy increased along with the decrease in temperature from 293to 77K because of deformation twinning and a decrease in stacking fault energy [13].After annealing at 850 C,its strength decreased because of grain coarsening [14].This alloy also exhibited fracture resistance at both high and low temperatures [15].The above studies indicate that the addition of Mn to an FCC high-entropy alloy may have a positive impact on its mechanical properties.So far,very little in the literature discusses the effect of Mn content variation on the high-entropy-alloy microstructure and*Corresponding author.E-mail address:ttshun@.tw (T.-T.Shun).Contents lists available at ScienceDirectMaterials Chemistry and Physicsjo urn al homepag e:/locate/matchemphys/10.1016/j.matchemphys.2017.07.0850254-0584/©2017Elsevier B.V.All rights reserved.Materials Chemistry and Physics xxx (2017)1e 6properties.In this work,the effects of Mn additions on the micro-structures and properties of Al 0.3CoCrFeNiMn x (x ¼0,0.1,and 0.3)high-entropy alloys are discussed.2.Material and methodsThe high-entropy alloys Al 0.3CoCrFeNiMn x (x ¼0,0.1,and 0.3,denoting Mn 0,Mn 0.1,and Mn 0.3,respectively)were produced through induction melting and casting in air.Elemental Al,Co,Cr,Fe,Ni,and Mn with a purity of more than 99wt%were used as raw materials.The ingot size was approximately 6cm Â8cm Â15cm.Samples cut from the ingot were used in microstructure analysis,hardness measurement,tensile test,and electrochemical corrosion test.The microstructure was examined by scanning electron mi-croscopy (SEM,HITACHI S-3400N)equipped with energy-dispersive spectrometry (EDS,BRUKER-XFLASH6/10).Aqua regia was employed as an etching solution for the SEM sample after polishing.The crystal structure was identi fied by X-ray diffrac-tometer (XRD,Bruker D8SSS)using Cu K a radiation at a scanning rate of 4 min À1and a 2q range of 20 e 100 .The hardness under a load of 300g for duration of 15s was measured by using a Vickers hardness tester (Future-Tech FR-300e).Five different positions were measured to calculate the average value and standard devi-ation.The plate-type subsize specimens (ASTM E8)were prepared for tensile test (Chun Yen CY-6040A4universal tensile testers)at a strain rate of 4Â10À4s À1at room temperature.The electro-chemical corrosion test was performed by potentiodynamic po-larization measurement using a 3.5%NaCl solution at room temperature.The test used a Biologic SP-150potentiostat at a scan rate of 5mV s À1,a segment of 1,a scan rang of À1to 1V,a frequency of 105e 10À2Hz,and an amplitude of 5mV.3.Results and discussion 3.1.Crystal structureFig.1shows the XRD patterns of the alloys Mn 0,Mn 0.1and Mn 0.3.All alloys exhibit a simple FCC ttice parameters of alloys calculated using Bragg formula [16]are 3.591,3.603and 3.611Å,respectively.The lattice parameter of the alloys increases slightly with an increase in the Mn content because the atomic radius of Mn (1.27Å)is smaller than those of Al (1.43Å)and Cr (1.28Å)but slightly larger than those of Co (1.25Å),Fe (1.26Å),and Ni (1.24Å).Therefore,addition of Mn to Al 0.3CoCrFeNi alloy induces the latticeexpansion.However,this lattice expansion is not large because the amount of Mn addition is only 0.1or 0.3mol.3.2.MicrostructureSEM images of Mn 0,Mn 0.1and Mn 0.3are displayed in Fig.2,and their chemical compositions as obtained by EDS analysis are listed in Table 1.All alloys exhibit a polycrystalline structure with grain size larger than 500m m.No secondary phase can be observed.These results con firm the XRD analyses results in Fig.1,which shows that all of the alloys comprise a simple FCC solid solution.In addition,some black dots inside the grains in Fig.2identi fied by EDS are mainly Al 2O 3inclusion,and the amount of inclusions in the three alloys is approximate (~2vol%).3.3.HardnessFig.3shows the hardness variation of the Al 0.3CoCrFeNiMn x alloys.The alloy hardness increases from HV141to HV156with the increase in Mn content to 6.8at%.The strengthening of the alloy can be attributed to two effects.One is solid-solution strengthening due to lattice distortion;the increase in lattice parameter of the alloy after Mn addition (section 3.1)explains this effect.The other effect is the reduction in stacking fault energy of the alloy,which makes cross-slipping of dislocations more dif ficult [17].This reduction upon the addition of the Mn may be deduced from the increase in mean electron hole number of each alloy,which is calculated by theformula ¼Pn i ¼1miN v i applied in superalloys [18].Here,is the average electron hole number of the alloy,n is the number of ele-ments in the alloy,mi is the atomic percentage of a particular element,and Nvi is the electron hole number of the element (Table 2).The calculated mean electron hole numbers of Mn 0,Mn 0.1and Mn 0.3are 2.44,2.47,and 2.52,respectively.Thus,the mean electron hole number increases upon addition of Mn.3.4.Tensile propertiesFig.4shows the engineering stress e strain curves obtained from tensile tests for Mn 0,Mn 0.1and Mn 0.3.Table 3summarizes data for the yield stress (s y ),ultimate tensile strength (s UTS ),elongation,and extent of work hardening (Ds ¼s UTS e s y ).Evidently,s y ,s UTS ,and Ds respectively increase from 119to 158MPa,from 295to 371MPa,and from 176to 213MPa with the increase in Mn content,while the elongation declines from 59%to 46%.The increase in s y is due to solid-solution strengthening,and the increase in s UTS results from the increase in work hardening due to the reduction in stacking fault energy.Engineering stress-strain curves of all of the alloys (Fig.4)have a yield point that is related to the homoge-neously spherical nanoprecipitates with the L12ordered structure reported in our previous study [19].These nanoprecipitates act as coherent GP zones,and the dislocation cutting through the particle results in a drop in the yield.Fig.5shows SEM images of the polished surface of Mn 0,Mn 0.1,and Mn 0.3under tension.Clearly,the deformation marks of the three alloys differ:that of the Mn 0has a wavy glide,and that of Mn 0.3has a planar glide,and that of Mn 0.1contains both types.The wavy glide created by a material with high stacking fault energy and narrowly separated partial dislocations facilitates cross-slipping of dislocations and results in lower work hardening dur-ing deformation.Conversely,the planar glide is a material with low stacking fault energy and widely separated partial dislocations,indicating more dif ficult for cross-slipping of dislocations and higher work hardening [20].The characteristics of the deformation mark on each alloy ’s surface thus con firm the effects of reductioninFig.1.XRD patterns of Mn 0,Mn 0.1,and Mn 0.3.S.-K.Wong et al./Materials Chemistry and Physics xxx (2017)1e 62Fig.2.SEM images of the alloys (a)Mn 0,(b)Mn 0.1,and (c)Mn 0.3.Table 1Chemical compositions (at%)of Al 0.3CoCrFeNiMn x alloys.AlloyAl Co Cr Fe Ni Mn Al 0.3CoCrFeNi6.923.323.423.323.1N/A Al 0.3CoCrFeNiMn 0.1 6.822.523.022.922.4 2.4Al 0.3CoCrFeNiMn 0.36.221.221.721.922.26.8Fig.3.Variation of hardness of Al 0.3CoCrFeNiMn x alloys.Table 2Electron hole number of individual element in alloy [17,18].AlCo Cr Fe Ni Mn Electron hole number2.661.714.662.660.663.66Fig.4.Tensile stress e strain curves of Mn 0,Mn 0.1,and Mn 0.3.S.-K.Wong et al./Materials Chemistry and Physics xxx (2017)1e 63stacking fault energy and the increase in strength upon addition of Mn to the alloys.3.5.Electrochemical corrosion propertiesPolarization curves of the alloys and of SUS304stainless steel are showed in Fig.6,and their electrochemical parameters are listed in Table 4.The corrosion potentials (E corr )of Mn 0,Mn 0.1,Mn 0.3and SUS304are À0.169,À0.172,À0.189,and À0.215V,respectively.This result indicates that the three high-entropy alloys have corrosion resistance better than that of SUS304.This result is due to the higher content of the anticorrosive elements Cr and Ni in the alloys as compared with that of SUS304.Fig.6also shows the po-larization curves of Mn 0,Mn 0.1and Mn 0.3mostly overlap,sug-gesting that the three alloys are almost identical in terms of corrosion resistance.The value of E corr (Table 4)indicates that the addition of Mn to the alloy leads to slight deterioration of the corrosion resistance.This deterioration is due to the increase in Mn content and the concomitant reduction of the Cr and Ni content.Table 3Yield stress (s y ),ultimate tensile strength (s UTS ),elongation,and extent of work hardening (Ds ¼s UTS e s y )of Mn 0,Mn 0.1and Mn 0.3.Alloy s y (MPa)s UTS (MPa)Elongation (%)Ds (MPa)Mn 011929559119Mn 0.114933649149Mn 0.315837146158Fig.5.SEM images of the polished surfaces of the alloys under tension:(a)Mn 0,(b)Mn 0.1,and (c)Mn 0.3.Fig.6.Polarization curves of Mn 0,Mn 0.1,and Mn 0.3alloys and of SUS304stainless steel.Table 4Electrochemical parameters of Mn 0,Mn 0.1,Mn 0.3and SUS304.Alloy E corr (V)I corr (mA/cm 2)E p (V)Mn 0À0.1690.3340.592Mn 0.1À0.1720.3250.579Mn 0.3À0.1890.3310.554SUS304À0.2150.3240.613S.-K.Wong et al./Materials Chemistry and Physics xxx (2017)1e 64The pitting potentials (E p )of Mn 0,Mn 0.1,Mn 0.3,and SUS304are 0.592,0.579,0.554,and 0.613V,respectively.The pitting re-sistances of the three alloys are thus lower than that of SUS304.Fig.7shows SEM images of the surface after electrochemical corrosion of Mn 0,Mn 0.1,Mn 0.3,and SUS304.The appearances of most pitting pores and inclusions in Mn 0to Mn 0.3alloys after testing are dark-gray square shape and black irregular shape,respectively.We suggest that these inclusions in alloys will some-what deteriorate the pitting resistance.However,this effect can be ignored in evaluation of pitting resistance among the present three high-entropy alloys due to their approximate inclusion parison of the number of pitting holes on each alloy agrees with the evaluation of pitting resistance from the E p value.The pitting resistance equivalent number (PRE N )of stainless steel is expressed as PRE N ¼Cr þ1.1Mo þ40.3N À1.3Mn [21].This equation is always used to predict the pitting resistance of the alloy in a Cl À-containing solution.Because Mn reduces the PRE N ,addi-tion of Mn to the alloy has an adverse effect on the pitting resis-tance.Calculated PRE N values of Mn 0,Mn 0.1,Mn 0.3,and SUS304are 12.09,10.20,6.65,and 18.06,respectively,which are in accordance with the experimental results.4.ConclusionsIn this study,the effect of Mn addition on the microstructures,mechanical properties,and electrochemical corrosion properties of Al 0.3CoCrFeNiMn x (x ¼0,0.1,0.3)high-entropy alloys were inves-tigated.The results show that all of the alloys exhibit an FCC polycrystalline structure.After the addition of Mn to Al 0.3CoCrFeNi alloy,the lattice parameter,hardness,yield stress,and ultimatetensile strength respectively increase from 3.591Åto 3.611Å,from HV141to HV156,from 119MPa to 158MPa,and from 295MPa to 371MPa.Solid-solution strengthening due to lattice distortion and work hardening due to reduction of stacking fault energy are two mechanisms behind the strengthening.The addition of Mn to the Al 0.3CoCrFeNi alloy leads to a slight deterioration of corrosion resistance.AcknowledgementThe authors would express gratitude for the financial support by the Ministry of Science and Technology of Taiwan under Grant No.MOST 104-2221-E-035-013.The authors also appreciate the Preci-sion Instrument Support Center of Feng Chia University in providing the fabrication and measurement facilities.References[1]J.W.Yeh,S.K.Chen,J.Y.Gan,T.S.Chin,T.T.Shun, C.H.Tsau,S.Y.Chang,Nanostructured high-entropy alloys with multiple principal elements:novel alloy design concepts and outcomes,Adv.Eng.Mater 6(5)(2004)299e 303.[2]Z.Wang,I.Baker,Z.Cai,S.Chen,J.D.Poplawsky,W.Guo,The effect of inter-stitial carbon on the mechanical properties and dislocation substructure evolution in Fe 40.4Ni 11.3Mn 34.8Al 7.5Cr 6high entropy alloys,Acta.Mater.120(2016)228e 239.[3]Z.Wu,M.Parish,H.Bei,Nano twin mediated plasticity in carbon containingFeNiCoCrMn high entropy alloys,pd.647(2015)815e 822.[4]Z.Li,C.C.Tasan,H.Springer,B.Gault,D.Raabe,Interstitial atoms enable jointtwinning and transformation induced plasticity in strong and ductile high-entropy alloys,Sci.Rep.7(40704)(2017)1e 7.[5]Z.Wang,I.Baker,Interstitial strengthening of a fcc FeNiMnAlCr high entropyalloy,Mater.Lett.180(2016)153e 156.[6]Y.Dong,K.Zhou,Y.Lu,X.Gao,T.Wang,T.Li,Effect of vanadium addition onthe microstructure and properties of AlCoCrFeNi high entropy alloy,Mater.Fig.7.SEM images of the surface after electrochemical corrosion of (a)Mn 0,(b)Mn 0.1,(c)Mn 0.3,and (d)SUS304.S.-K.Wong et al./Materials Chemistry and Physics xxx (2017)1e 65Des.57(2014)67e72.[7]Y.J.Zhou,Y.Zhang,F.J.Wang,Y.L.Wang,G.L.Chen,Effect of Cu addition on themicrostructure and mechanical properties of AlCoCrFeNiTi0.5solid-solution alloy,pd.466(2008)201e204.[8]J.M.Zhu,H.M.Fu,H.F.Zhang,A.M.Wang,H.Lia,Z.Q.Hua,Microstructures andcompressive properties of multicomponent AlCoCrFeNiMo x alloys,Mater.Sci.Eng.A527(2010)6975e6979.[9] C.F.Lee,T.T.Shun,Effect of Fe content on microstructure and mechanicalproperties of Al0.5CoCrFe x NiTi0.5high entropy alloys,Mater.Charact.114 (2016)179e184.[10]I.S.Wani,T.Bhattacharjee,S.Sheikh,P.P.Bhattacharjee,S.Guo,N.Tsuji,Tailoring nanostructures and mechanical properties of AlCoCrFeNi2.1eutectic high entropy alloy using thermos mechanical processing,Mat.Sci.Eng.A675 (2016)99e109.[11]G.E.Totten,L.Xie,K.Funatani,Handbook of Mechanical Alloy Design,MarcelDekker,Inc.,New York,2004.[12] B.S.Li,Y.P.Wang,M.X.Ren,C.Yang,H.Z.Fu,Effects of Mn,Ti and V on themicrostructure and properties of AlCrFeCoNiCu high entropy alloy,Mat.Sci.Eng.A498(2008)482e486.[13] F.Otto,A.Dlouhý,Ch Somsen,H.Bei,G.Eggeler,E.P.George,The influences oftemperature and microstructure on the tensile properties of a CoCrFeMnNi high-entropy alloy,Acta.Mater.61(2013)5743e5755.[14]W.H.Liu,Y.Wu,J.Y.He,T.G.Nieh,Z.P.Lu,Grain growth and the Hall-Petchrelationship in a high-entropy FeCrNiCoMn alloy,Scr.Mater.68(2013) 526e529.[15] B.Gludovatz,et al.,A fracture-resistant high-entropy alloy for cryogenic ap-plications,Sci.345(2014)1153e1158.[16] B.D.Cullity,S.R.Stock,Elements of X-ray Diffraction,third ed.,Prentice-Hall,Inc.,New Jersey,2001.[17]W.F.Smith,Structure and Properties of Engineering Alloys,second ed.,McGraw-Hill,Inc.,1993.[18] C.T.Sims,N.S.Stoloff,W.C.Hagel,Superalloys II,John Wiley&Sons,Inc.,1987.[19]T.T.Shun,Y.C.Du,Microstructure and tensile behaviors of FCC Al0.3CoCrFeNihigh entropy alloy,pd.479(2009)157e160.[20]R.W.Hertzberg,Deformation and Fracture Mechanics of Engineering Mate-rials,second ed.,John Wiley&Sons,Inc.,1983.[21]M.O.Speidel,R.M.Pedrazzoli,High nitrogen stainless steels in chloride solu-tions,Mater.Perform.31(9)(1992)59e61.S.-K.Wong et al./Materials Chemistry and Physics xxx(2017)1e6 6。
材料科技英语句子翻译重点

材料科技英语考试句子翻译复习(1-5,1/5)1. Material science involves investigating the relationship that exists between the structures and properties of materials. In contrast,“material engineering”is on the basis of these structure-property correlations,designing or engineering the structure of a material to produce a predetermined set of properties材料科学是研究材料的结构和性能之间存在的关系,相反,材料工程是在这些结构性能相互关系的基础上,设计和构建材料的结构来实现一系列的预定的性能。
2. Virtually all important properties of solid materials may be grouped into six different categories:mechanical,electrical,thermal,magnetic,optical,and deteriorative实际上,固体材料的所有重要材料可以被分成六个目录,力学,热学,磁学,光学和失效。
3. In addition to structure and properties, two other important components are involved in the science and engineering of materials,namely“processing”and “performance”.除了结构和性质,还有两个重要的材料科学与工程的性质是材料加工材料和性能。
超声纳米晶表面改性对选区激光熔化316L_不锈钢微观结构和力学性能的影响

第53卷第5期表面技术2024年3月SURFACE TECHNOLOGY·137·表面强化技术超声纳米晶表面改性对选区激光熔化316L不锈钢微观结构和力学性能的影响彭兰,张宇,高乐,叶一璇,叶畅*(华中科技大学,武汉 430074)摘要:目的改善选区激光熔化(Selective laser melting,SLM)316L不锈钢的表面完整性和力学性能。
方法采用超声纳米晶表面改性(Ultrasonic Nanocrystal Surface Modification,UNSM)这一新兴表面塑性变形方法对SLM 316L不锈钢进行超声冲击强化,利用维氏硬度计、扫描电镜、白光干涉仪、EBSD、XRD等对处理前后材料的表面完整性、微观组织演变和塑性变形行为进行表征和分析。
结果经过UNSM处理后,SLM 316L不锈钢的微观缺陷明显减少,初始未熔合孔隙发生闭合,表面粗糙度Ra由5.374 μm降至0.510 μm,表面硬度从230HV增至461.16HV;同时,材料表层发生了剧烈的塑性变形,形变诱导材料微观组织从γ相向α相转变,微观结构由初始不规则柱状粗晶转变为等轴状细晶。
从EBSD表征结果可知,在材料表面形成了深度约为20 μm的梯度纳米晶,材料内部存在明显的不均匀变形;与初始SLM试样相比,通过UNSM 处理在材料表面引入了最大为932 MPa的残余压应力。
结论超声纳米晶表面改性能够显著改善SLM 316L 不锈钢的表面完整性,形成较深的晶粒细化层和残余应力硬化层,从而有效提高其耐腐蚀性和疲劳抗性,是一项有前景的SLM后处理技术。
关键词:超声纳米晶表面改性;SLM 316L不锈钢;微观结构;残余应力;塑性变形机理中图分类号:TG665;TG142.71 文献标志码:A 文章编号:1001-3660(2024)05-0137-12DOI:10.16490/ki.issn.1001-3660.2024.05.014Effect of Ultrasonic Nanocrystal Surface Modification on Microstructure and Mechanical Properties of SLM 316L Stainless SteelPENG Lan, ZHANG Yu, GAO Le, YE Yixuan, YE Chang*(Huazhong University of Science and Technology, Wuhan 430074, China)ABSTRACT: Metal powder additive manufacturing (AM) technologies, such as selective laser melting (SLM), have attracted considerable interest owing to their near-net forming characteristic and layer-by-layer building-up strategy, which allows overcoming the constraints of traditional manufacturing technology, achieving complex components in a short time of mass customization. However, the SLM process-induced micro-defects (i.e. pores, lack-of-fusion, and undesired microstructures) will result in not only poor surface finish and interior thermal cracks but also more dispersion收稿日期:2023-03-24;修订日期:2023-06-14Received:2023-03-24;Revised:2023-06-14基金项目:国家自然科学基金(52075200)Fund:National Natural Science Foundation of China (52075200)引文格式:彭兰, 张宇, 高乐, 等. 超声纳米晶表面改性对选区激光熔化316L不锈钢微观结构和力学性能的影响[J]. 表面技术, 2024, 53(5): 137-148.PENG Lan, ZHANG Yu, GAO Le, et al. Effect of Ultrasonic Nanocrystal Surface Modification on Microstructure and Mechanical Properties of SLM 316L Stainless Steel[J]. Surface Technology, 2024, 53(5): 137-148.*通信作者(Corresponding author)·138·表面技术 2024年3月of mechanical properties. Therefore, for a more homogenized microstructure and smaller material anisotropy, a novel surface strengthening method of severe surface plastic deformation, ultrasonic nanocrystalline surface modification (UNSM) was applied to improve the surface integrity and mechanical properties of SLM 316L stainless steel in this study.A medium size laser powder bed fusion (LPBF 271 Series device from Farsoon Technologies Tech Co., Ltd.) wasused to fabricate the plate specimens with 316L stainless steel powder. The SLM-processed samples were fabricated using the optimized processing parameters with a laser power of 400 W, hatch spacing of 0.11 μm, a laser scan speed of 1 250 mm/s, and a layer thickness of 60 μm. Bidirectional laser scanning with a scan rotation of 67° for every layer was performed during building. An annealing heat treatment at 900 ℃for 2 h was conducted on the as-received SLM 316L stainless steel plate, followed by furnace cooling to room temperature. The SLM 316L stainless steel plate used in this investigation was 40 mm×20 mm×4 mm in size. For improving the strengthening efficacy, optimized UNSM process parameters were used in the current work: an ultrasonic frequency of 20 kHz, a WC (tungsten carbide) tip with a diameter of 2.4 mm, a static load of 50 N, an ultrasonic amplitude of 30 µm, a scanning speed of 500 mm/min, and a feed rate of 10 µm.The surface integrity, microstructure evolution, and plastic deformation behavior of the material before and after UNSM treatment were systematically characterized and analyzed through Vickers indentation, a scanning electron microscope (SEM), a white light interferometer, electron backscatter diffraction (EBSD), and x-ray diffraction (XRD).The result showed that the micro defects of SLM 316L stainless steel were significantly reduced. SLM's initial LOF defects were diminished under the high-frequency ultrasonic load. The surface roughness Ra decreased from 5.374 μm to 0.510 μm, and the surface hardness increased from 230HV to 461.16HV. Severe plastic deformation (SPD) occurred on the surface layer of the material, which induced the transformation of the microscopic structure from γ to α phase. The crystal microstructure was also refined from the initial irregular columnar coarse crystal to fine equiaxed crystal. As a result of local uneven plastic deformation in the UNSM process, a depth 20 μm gradient nanocrystal was captured through the result of EBSD analysis. Thus, compared with the initial SLM specimen, the UNSM treatment produced a maximum residual compressive stress of 932 MPa on the surface of the material. The improvement of the surface integrity, formation of deeper grain refinement layer, and residual stress hardening layer of SLM 316L after UNSM treatment successfully demonstrates that UNSM is a promising post-processing surface treatment technology for SLM metallic materials.KEY WORDS: UNSM; SLM 316L stainless steel; microstructure; residual stress; plastic deformation mechanism在各牌号不锈钢中,316L不锈钢的含碳量较低,且其中添加了质量分数为2%~3%的Mo元素,因此它在耐腐蚀性能方面表现优异,可以应用于金属易腐蚀的恶劣环境。
Mo元素对Al0.3CrFeCoNiMox高熵合金组织结构、力学性能和腐蚀行为的影响

第 1 期第 118-127 页材料工程Vol.52Jan. 2024Journal of Materials EngineeringNo.1pp.118-127第 52 卷2024 年 1 月Mo 元素对Al 0.3CrFeCoNiMo x高熵合金组织结构、力学性能和腐蚀行为的影响Effects of Mo element on microstructure ,mechanical properties and corrosion behavior of Al 0.3CrFeCoNiMo x high -entropy alloys范世超,廖振龙,李柔珊,逄淑杰*(北京航空航天大学 材料科学与工程学院,北京100191)FAN Shichao ,LIAO Zhenlong ,LI Roushan ,PANG Shujie *(School of Materials Science and Engineering ,BeihangUniversity ,Beijing 100191,China )摘要:为提高Al -Cr -Fe -Co -Ni 系高熵合金的力学性能和耐腐蚀性能,研究Mo 元素对 Al 0.3CrFeCoNiMo x (x = 0.2,0.4,0.6,0.8,摩尔比,下同)高熵合金的组织结构、力学性能及腐蚀行为的影响。
结果表明:随着Mo 元素含量的提高,合金由FCC 相(x =0.2)转变为FCC+σ双相结构(x =0.4~0.8)。
当Mo 元素含量由x =0.2增加到x =0.8时,合金的压缩屈服强度和硬度分别由304 MPa 和214HV 提高到1192 MPa 和513HV ,塑性应变由>50%降低到5.2%,这主要是由于固溶强化作用和σ相含量增加。
该合金系中,Al 0.3CrFeCoNiMo 0.4和Al 0.3CrFeCoNiMo 0.6合金具有较高的屈服强度(571~776 MPa )和较好的塑性(塑性应变10.3%~23.8%)。
放电等离子体烧结Al-4.5Cu合金的组织与性能
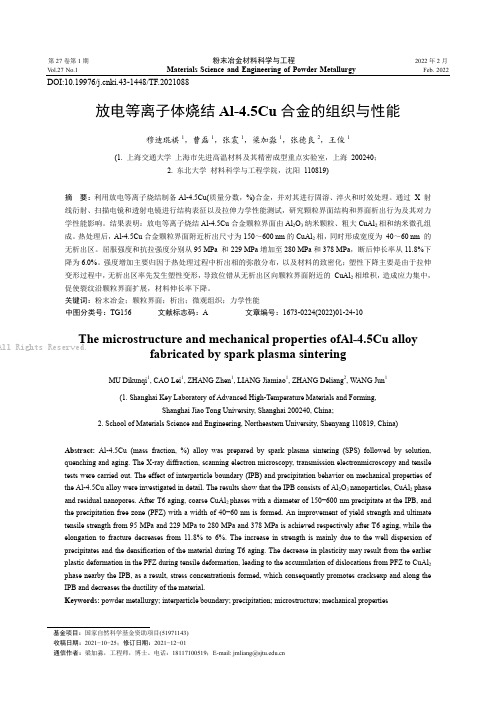
第27卷第1期粉末冶金材料科学与工程2022年2月V ol.27 No.1 Materials Science and Engineering of Powder Metallurgy Feb. 2022DOI:10.19976/ki.43-1448/TF.2021088放电等离子体烧结Al-4.5Cu合金的组织与性能穆迪琨祺1,曹磊1,张震1,梁加淼1,张德良2,王俊1(1. 上海交通大学上海市先进高温材料及其精密成型重点实验室,上海200240;2. 东北大学材料科学与工程学院,沈阳110819)摘要:利用放电等离子烧结制备Al-4.5Cu(质量分数,%)合金,并对其进行固溶、淬火和时效处理。
通过X 射线衍射、扫描电镜和透射电镜进行结构表征以及拉伸力学性能测试,研究颗粒界面结构和界面析出行为及其对力学性能影响。
结果表明:放电等离子烧结Al-4.5Cu合金颗粒界面由Al2O3纳米颗粒、粗大CuAl2相和纳米微孔组成。
热处理后,Al-4.5Cu合金颗粒界面附近析出尺寸为150~600 nm的CuAl2相,同时形成宽度为40~60 nm 的无析出区。
屈服强度和抗拉强度分别从95 MPa 和229 MPa增加至280 MPa和378 MPa,断后伸长率从11.8%下降为6.0%。
强度增加主要归因于热处理过程中析出相的弥散分布,以及材料的致密化;塑性下降主要是由于拉伸变形过程中,无析出区率先发生塑性变形,导致位错从无析出区向颗粒界面附近的CuAl2相堆积,造成应力集中,促使裂纹沿颗粒界面扩展,材料伸长率下降。
关键词:粉末冶金;颗粒界面;析出;微观组织;力学性能中图分类号:TG156文献标志码:A 文章编号:1673-0224(2022)01-24-10The microstructure and mechanical properties ofAl-4.5Cu alloyAll Rights Reserved.fabricated by spark plasma sinteringMU Dikunqi1, CAO Lei1, ZHANG Zhen1, LIANG Jiamiao1, ZHANG Deliang2, WANG Jun1(1. Shanghai Key Laboratory of Advanced High-Temperature Materials and Forming,Shanghai Jiao Tong University, Shanghai 200240, China;2. School of Materials Science and Engineering, Northeastern University, Shenyang 110819, China)Abstract:Al-4.5Cu (mass fraction, %) alloy was prepared by spark plasma sintering (SPS) followed by solution,quenching and aging. The X-ray diffraction, scanning electron microscopy, transmission electronmicroscopy and tensiletests were carried out. The effect of interparticle boundary (IPB) and precipitation behavior on mechanical properties ofthe Al-4.5Cu alloy were investigated in detail. The results show that the IPB consists of Al2O3 nanoparticles, CuAl2 phaseand residual nanopores. After T6 aging, coarse CuAl2 phases with a diameter of 150−600 nm precipitate at the IPB, andthe precipitation free zone (PFZ) with a width of 40−60 nm is formed. An improvement of yield strength and ultimatetensile strength from 95 MPa and 229 MPa to 280 MPa and 378 MPa is achieved respectively after T6 aging, while theelongation to fracture decreases from 11.8% to 6%. The increase in strength is mainly due to the well dispersion ofprecipitates and the densification of the material during T6 aging. The decrease in plasticity may result from the earlierplastic deformation in the PFZ during tensile deformation, leading to the accumulation of dislocations from PFZ to CuAl2phase nearby the IPB, as a result, stress concentrationis formed, which consequently promotes cracksexp and along theIPB and decreases the ductility of the material.Keywords: powder metallurgy; interparticle boundary; precipitation; microstructure; mechanical properties基金项目:国家自然科学基金资助项目(51971143)收稿日期:2021−10−25;修订日期:2021−12−01通信作者:梁加淼,工程师,博士。
Microstructures and Dielectric Properties
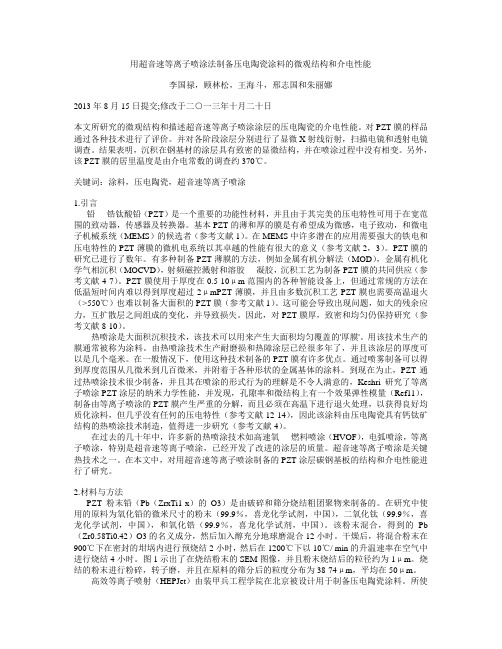
用超音速等离子喷涂法制备压电陶瓷涂料的微观结构和介电性能李国禄,顾林松,王海斗,邢志国和朱丽娜2013年8月15日提交;修改于二○一三年十月二十日本文所研究的微观结构和描述超音速等离子喷涂涂层的压电陶瓷的介电性能。
对PZT膜的样品通过各种技术进行了评价。
并对各阶段涂层分别进行了显微X射线衍射,扫描电镜和透射电镜调查。
结果表明,沉积在钢基材的涂层具有致密的显微结构,并在喷涂过程中没有相变。
另外,该PZT膜的居里温度是由介电常数的调查约370℃。
关键词:涂料,压电陶瓷,超音速等离子喷涂1.引言铅- 锆钛酸铅(PZT)是一个重要的功能性材料,并且由于其完美的压电特性可用于在宽范围的致动器,传感器及转换器。
基本PZT的薄和厚的膜是有希望成为微感,电子致动,和微电子机械系统(MEMS)的候选者(参考文献1)。
在MEMS中许多潜在的应用需要强大的铁电和压电特性的PZT薄膜的微机电系统以其卓越的性能有很大的意义(参考文献2,3)。
PZT膜的研究已进行了数年。
有多种制备PZT薄膜的方法,例如金属有机分解法(MOD),金属有机化学气相沉积(MOCVD),射频磁控溅射和溶胶- 凝胶,沉积工艺为制备PZT膜的共同供应(参考文献4-7)。
PZT膜使用于厚度在0.5-10μm范围内的各种智能设备上,但通过常规的方法在低温短时间内难以得到厚度超过2μmPZT薄膜,并且由多数沉积工艺PZT膜也需要高温退火(>550℃)也难以制备大面积的PZT膜(参考文献1)。
这可能会导致出现问题,如大的残余应力,互扩散层之间组成的变化,并导致损失。
因此,对PZT膜厚,致密和均匀仍保持研究(参考文献8-10)。
热喷涂是大面积沉积技术,该技术可以用来产生大面积均匀覆盖的'厚膜'。
用该技术生产的膜通常被称为涂料。
由热喷涂技术生产耐磨损和热障涂层已经很多年了,并且该涂层的厚度可以是几个毫米。
在一般情况下,使用这种技术制备的PZT膜有许多优点。
Microstructure and thermal properties of copper matrix composites译文

HUNAN UNIVERSITY 论文翻译译文论文题目不连续镀铬石墨纤维增强铜基复合材料的微观结构和热力学性能的研究学生姓名张成智学生学号20111402128专业班级材料成型及控制工程2011级1班学院名称材料科学与工程学院指导老师刘金水学院院长陈江华二〇一五年四月不连续镀铬石墨纤维增强铜基复合材料的微观结构和热力学性能的研究摘要通过化学气相沉积(CVD)技术在不连续中间相沥青基石墨纤维上涂上一层金属铬,并用热压烧结技术制造出铜基涂铬石墨纤维增强的复合材料。
它们的微观结构和热力学性能包括热传导率和热膨胀系数(CTE)。
结果显示由于制造过程的影响,纤维在铜基2-D上随机排列,这就导致了复合材料热力学性能的各向异性。
Cr涂层会与石墨纤维发生反应形成一层薄而连续的Cr3C2层,这个Cr3C2层能在纤维和Cu基建立良好的冶金界面结合,这有利于提高热力学性能和降低复合材料的CTE。
纤维含量在35-50vol%的复合材料,它能达到98%以上真实密度,380-412W/mK面内的热传导率和6.1-9.4×10-6/K的面内CET。
由于这种复合材料具有高的热力学性能、低的CET及好的机械加工性能,所以它在散热片材料的应用和开发中具有很好前景的复合材料。
关键词:金属及复合材料;微观结构;热传导;热膨胀Microstructure and thermal properties of copper matrix composites reinforced by chromium-coated discontinuous graphite fibersAbstractDiscontinuous mesophase pitch-based graphite fibers were coated with chromium via chemical vapor deposition technique and Cr-coated graphite fiber/Cu composites were fabricated by hot-pressing sintering. Their microstructure and thermal properties,including thermal conductivity and coefficient of thermal expansion (CTE),were investigated. Results show that the fabrication process led to the fibers with a 2-D random arrangement in Cu matrix,resulting in anisotropic thermal properties of the composites. The Cr coating reacted with graphite fiber and formed a thin and continuous Cr3C2 layer. This Cr3C2 layer established a good metallurgical interfacial bonding between the fiber and Cu matrix,which helps to enhance the thermal properties and to reduce the CTEs of the composites. The composites with 35-50 vol% content of fibers achieved the relative densities of >98%,the in-plane thermal conductivities of 380-412 W/mK and the in-plane CTEs of 6.1-9.4×10-6/K. Due to the high thermal conductivity,low CTE and good machinability,the composites are promising materials for heat sink applications.Keywords:Metal matrix composites;Microstructure;Heat conduction;Thermal expansion1 介绍从以往任何时候都更小,更强烈地散热的电子散热设备已成为电子行业热议的话题。
材料科学与工程专业英语1-18单元课后翻译答案
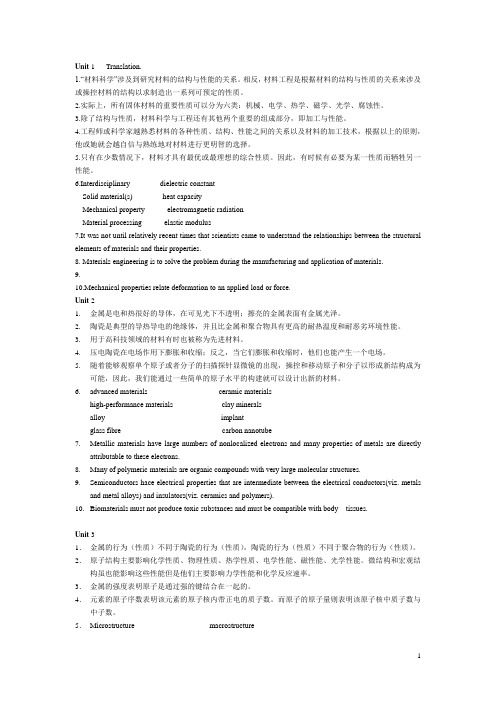
Unit 1 Translation.1.“材料科学”涉及到研究材料的结构与性能的关系。
相反,材料工程是根据材料的结构与性质的关系来涉及或操控材料的结构以求制造出一系列可预定的性质。
2.实际上,所有固体材料的重要性质可以分为六类:机械、电学、热学、磁学、光学、腐蚀性。
3.除了结构与性质,材料科学与工程还有其他两个重要的组成部分,即加工与性能。
4.工程师或科学家越熟悉材料的各种性质、结构、性能之间的关系以及材料的加工技术,根据以上的原则,他或她就会越自信与熟练地对材料进行更明智的选择。
5.只有在少数情况下,材料才具有最优或最理想的综合性质。
因此,有时候有必要为某一性质而牺牲另一性能。
6.Interdisciplinary dielectric constantSolid material(s) heat capacityMechanical property electromagnetic radiationMaterial processing elastic modulus7.It was not until relatively recent times that scientists came to understand the relationships between the structural elements of materials and their properties.8. Materials engineering is to solve the problem during the manufacturing and application of materials.9.10.Mechanical properties relate deformation to an applied load or force.Unit 21.金属是电和热很好的导体,在可见光下不透明;擦亮的金属表面有金属光泽。
烧成温度对钙长石隔热耐火材料显微结构与性能的影响

第43卷第3期2024年3月硅㊀酸㊀盐㊀通㊀报BULLETIN OF THE CHINESE CERAMIC SOCIETY Vol.43㊀No.3March,2024烧成温度对钙长石隔热耐火材料显微结构与性能的影响陈㊀曦,刘㊀莹,鄢㊀文(武汉科技大学省部共建耐火材料与冶金国家重点实验室,武汉㊀430081)摘要:本工作以矾土水泥与黏土为原料,采用发泡法制备了钙长石隔热耐火材料,通过XRD㊁SEM 和导热仪等分析了烧成温度(1110~1310ħ)对钙长石隔热耐火材料显微结构与性能的影响,并研究了该种钙长石隔热耐火材料的合成机理㊂结果表明:当烧成温度为1110~1160ħ时,体系内开始生成钙长石,但原料微颗粒间的反应并不完全,生成的液相含量较少,强度较低;当烧成温度为1210~1260ħ时,液相含量增多,钙长石的生成速度加快,强度增大,试样具有较低导热系数;当烧成温度为1310ħ时,液相含量迅速增多,线收缩率迅速增大,试样具有较大强度,但体积密度大幅增长,气孔率降低㊂在1260ħ时,制得了体积密度为0.53g /cm 3㊁气孔率为82.6%㊁抗折强度为1.15MPa㊁耐压强度为1.99MPa㊁800ħ导热系数为0.101W /(m㊃K)的钙长石隔热耐火材料㊂关键词:钙长石;发泡法;烧成温度;隔热;玻璃相;孔径分布中图分类号:TB321㊀㊀文献标志码:A ㊀㊀文章编号:1001-1625(2024)03-1123-10Effect of Sintering Temperature on Microstructure and Properties of Anorthite Insulation RefractoryCHEN Xi ,LIU Ying ,YAN Wen(The State Key Laboratory of Refractories and Metallurgy,Wuhan University of Science and Technology,Wuhan 430081,China)Abstract :In this work,bauxite cement and clay were used as raw materials to prepare anorthite insulation refractory by foaming method.On the basis of analyzing the effect of sintering temperature (1110~1310ħ)on the microstructure and properties of anorthite insulation refractory by XRD,SEM and thermal conductivity meter,the synthesis mechanism of anorthite insulation was studied.The results show that when the sintering temperature is between 1110ħand 1160ħ,anorthite begins to form,but the reactions between raw materials are not complete,the liquid phase content is less and the strength is lower.When the sintering temperature is between 1210ħand 1260ħ,the liquid phase content increases,the rate of anorthite formation was accelerates,the strength increases,and the samples have a low thermal conductivity.When the sintering temperature is 1310ħ,the liquid phase content increases rapidly,the linear shrinkage rate increases rapidly,and the samples have higher strength,but the bulk density increases significantly and the porosity decreases.At 1260ħ,the anorthite insulation refractory is prepared,with bulk density of 0.53g /cm 3,porosity of 82.6%,flexural strength of 1.15MPa,compressive strength of 1.99MPa,and thermal conductivity of 0.101W/(m㊃K)at 800ħ.Key words :anorthite;foaming method;sintering temperature;insulation;glass phase;pore size distribution 收稿日期:2023-10-19;修订日期:2023-11-10作者简介:陈㊀曦(1999 ),男,硕士研究生㊂主要从事制备钙长石隔热耐火材料的研究㊂E-mail:535394769@通信作者:鄢㊀文,博士,教授㊂E-mail:yanwen@ 0㊀引㊀言隔热耐火材料具有气孔率高㊁导热系数低等优点,常被用作高温窑炉的保温衬[1],对减少高温窑炉热量损失和降低高温工业能耗起着重要作用[2]㊂钙长石具有体积密度小㊁导热系数低等优点,以其为主要成分的多孔陶瓷是一种良好的隔热材料[3-4]㊂因此,为了降低高温窑炉耐火内衬的散热损失,钙长石隔热耐火材1124㊀耐火材料硅酸盐通报㊀㊀㊀㊀㊀㊀第43卷料的研究受到越来越多的关注[5-8]㊂目前钙长石隔热耐火材料的常见制备方法有添加造孔剂法[9]㊁泡沫注凝法[10]以及发泡法[11]等㊂韩耀[12]使用泡沫注凝法制备的钙长石隔热耐火材料气孔率为83%,抗压强度为1.65MPa,但其工艺复杂且成本较高㊂顾幸勇等[13]以煅烧黏土和硅灰石为主要原料,通过添加造孔剂法制备了体积密度为0.52g/cm3㊁抗压强度为0.13MPa㊁导热系数(室温)为0.16W/(m㊃K)的钙长石隔热耐火材料,但气孔分布不均匀,容易因结构缺陷造成试样开裂[13],并且造孔剂为可燃物,污染环境㊂相比之下,发泡法工艺简单,制品气孔率高,且不会因造孔剂烧失造成二次污染㊂倪文等[14]以煅烧煤矸石和蓝晶石为主要原料,利用发泡法制备了体积密度为0.47g/cm3㊁抗折强度为0.92MPa㊁导热系数(800ħ)为0.168W/(m㊃K)的钙长石隔热耐火材料㊂耿浩洋等[15]以高岭土和鲁山石为主要原料,利用发泡法制备了体积密度为0.5g/cm3㊁抗压强度为1.25MPa㊁平均孔径为700μm的钙长石隔热耐火材料㊂然而,现有发泡法产生的气泡大小不均匀,易因气孔合并产生较大气泡,且制备的钙长石隔热耐火材料无法同时满足更高强度和更低导热的工业化需求㊂研究[16]表明,浆体的流动性和凝固时间对发泡效果和气孔尺寸具有重要影响,引入铝酸盐水泥不仅有利于解决浆料的凝固和硬化问题,且能提高生坯的强度,便于脱模㊂刘强等[17]引入23%(质量分数)的铝酸盐水泥作为增强剂,制备了体积密度低于0.55g/cm3的钙长石轻质隔热砖,不仅解决了浆体流动性的问题,同时提高了生坯强度㊂除了增大气孔率㊁降低气孔孔径来降低导热系数外,控制材料内部玻璃相含量和黏度也是降低导热系数的有效手段[18-20]㊂本试验以矾土水泥和黏土为原料,通过发泡法制备钙长石隔热耐火材料㊂一方面利用水泥的快硬性促进坯体成型,有效阻止发泡后小气孔合并形成大气孔,减小气孔孔径;另一方面,利用矾土水泥和黏土特定组成,在设计钙长石为主晶相的基础上,控制其玻璃相的含量与黏度,在促进骨架烧结的同时,大幅降低导热系数㊂通过研究烧成温度对钙长石隔热耐火材料显微结构与性能的影响,提出合成机理㊂1㊀实㊀验1.1㊀原㊀料主要原料为黏土(河南偃师光明高科技有限公司)和矾土水泥(郑州嘉耐特种铝酸盐有限公司),主要化学组成如表1所示,发泡剂为十二烷基硫酸钠(SDS,国药集团化学试剂有限公司),稳泡剂为羧甲基纤维素(CMC,国药集团化学试剂有限公司),减水剂为三聚磷酸钠(STP,国药集团化学试剂有限公司)㊂图1为黏土和水泥的XRD谱㊂由图1可知,水泥的物相组成为一铝酸钙㊁二铝酸钙㊁铝酸三钙和钙铝黄长石,黏土的物相组成为高岭石㊁微斜长石和铁云母㊂图2为黏土的粒度分布和累积分布,颗粒尺寸呈单峰分布,粒径峰为4.08μm,中位粒径为4.41μm㊂表1㊀原料的主要化学组成Table1㊀Main chemical composition of raw materialsRaw material Mass fraction/%Al2O3SiO2Fe2O3CaO MgO K2O Na2O TiO2LOI Clay28.0245.76 1.490.110.150.170.22 1.9121.52 Alumina cement53.87 5.39 1.3532.77 1.230.230.42 2.32 2.101.2㊀样品制备将矾土水泥和黏土按质量比47ʒ53进行配料㊂将配好的原料置于聚氨酯混料桶中以刚玉球为介质混料2h后,将三聚磷酸钠(STP,11g/L)㊁十二烷基硫酸钠(SDS,6.5g/L)和羧甲基纤维素(CMC,4g/L)溶于水后制得溶液,其中m(原料)ʒm(水)=60ʒ40,用家用打蛋器搅拌发泡获得泡沫浆料㊂将发泡后的浆体迅速倒入150mmˑ40mmˑ40mm的长条模具和ϕ50mmˑH50mm的圆柱模具中,分别制得长条样和圆柱样,在室温(大于30ħ)下养护24h后,再放入50ħ烘箱养护12h后进行脱模㊂将脱模后的试样经110ħˑ24h烘干后,置于高温炉中,以5ħ/min的升温速率升温至1000ħ,再以3ħ/min的升温速率升温至目标温度并保温3h,目标温度分别为1110㊁1160㊁1210㊁1260㊁1310ħ,随炉冷却至室温㊂第3期陈㊀曦等:烧成温度对钙长石隔热耐火材料显微结构与性能的影响1125㊀图1㊀矾土水泥和黏土的XRD 谱Fig.1㊀XRD patterns of bauxite cement andclay 图2㊀黏土的粒度分布和累积分布曲线Fig.2㊀Particle size distribution and cumulative distribution curves ofclay 图3㊀经不同温度烧后各试样的XRD 谱Fig.3㊀XRD patterns of each sample after sintered at different temperatures 1.3㊀样品的表征使用荷兰X Pert ProPhilips 的X 射线衍射仪对烧后试样的物相组成进行分析㊂利用日本JEOL 公司的扫描电子显微镜(SEM,JSM-6610)和能谱仪(EDS,QUANTAX200-30,BRUKER,Germany)测定试样的显微结构和微区成分,并使用Image pro 6.0图像分析软件处理SEM 照片,以测定孔径大小及分布状态㊂使用真密度测试仪测定烧后试样的真密度,并计算得到试样的总气孔率㊂根据‘耐火材料常温抗折强度试验方法“(GB /T 3001 2007)和‘耐火材料常温耐压强度试验方法“(GB /T 5072 2008)分别测试试样在常温下的抗折强度和耐压强度㊂根据‘耐火材料导热系数试验方法“(YB /T 4130 2005),使用水流平板法测定圆盘试样的导热系数㊂使用FactSage 6.2软件中的Equilib模块和Viscosity 模块,计算试样在高温下的液相含量和液相黏度㊂2㊀结果与讨论2.1㊀物相组成图3给出了经不同温度烧后各试样的XRD 谱㊂当烧成温度为1110ħ时,烧后试样的主要物相为钙铝黄长石㊁石英以及二铝酸钙㊂当烧成温度为1160ħ时,主要物相为刚玉㊁钙长石和钙铝黄长石㊂当烧成温度为1210ħ时,主要物相为钙长石和钙铝黄长石㊂当烧成温度升高至1260ħ时,仅有钙长石一种物相㊂1126㊀耐火材料硅酸盐通报㊀㊀㊀㊀㊀㊀第43卷而当烧成温度继续升高到1310ħ时,除了钙长石,还出现少量钙铝榴石和红铝透辉石㊂2.2㊀显微结构图4~6分别给出了经1110㊁1160㊁1210ħ烧后试样的抛光面与断口处的SEM照片㊂其中C2AS为钙铝黄长石,CAS2为钙长石,CA2为二铝酸钙㊂图4(a)㊁图5(a)和图6(a)分别为三种温度烧后试样抛光面的SEM照片㊂由图可知:当烧成温度为1110ħ时,试样中存在两种类型的气孔,分别是孔壁内的小气孔和发泡所产生的孔壁间大气孔,且孔壁之间存在较多未反应完全的原料大颗粒;当烧成温度为1160ħ时,孔壁内的原料大颗粒逐渐减少,小气孔逐渐增多,并且孔壁间大气孔孔径增大;当烧成温度为1210ħ时,孔壁厚度减小,孔壁内仅存在少量未反应完全的原料大颗粒,孔壁间大气孔孔径继续增大㊂图4(b)㊁(c),图5(b)㊁(c)和图6(b)㊁(c)为经三种温度烧后试样断口处的SEM照片㊂当烧成温度为1110ħ时,原料颗粒之间连接杂乱,能明显地看到未反应的原料大颗粒,并且颗粒表面存在大量细小颗粒以及少量的片状钙铝黄长石,结合XRD分析可知细小颗粒为铝酸钙晶粒㊂当烧成温度为1160ħ时,颗粒的尺寸明显减小,表面的晶粒尺寸减小,细小的片状钙铝黄长石尺寸增大㊂当烧成温度为1210ħ时,钙长石反应基本完成,但其尺寸较小,发育不完全,在颗粒表面仍能看见团絮状的小颗粒堆积,根据XRD和EDS结果可知,絮状的小颗粒为未发育成型的钙铝黄长石㊂图4㊀经1110ħ烧后试样的SEM照片Fig.4㊀SEM images of samples sintered at1110ħ图5㊀经1160ħ烧后试样的SEM照片Fig.5㊀SEM images of samples sintered at1160ħ图7给出了经1260ħ烧后试样的抛光面与断口处的SEM照片㊂图7(a)为1260ħ烧后试样抛光面的SEM照片,当烧成温度为1260ħ时,孔壁内的原料反应完全,孔壁厚度进一步减小,孔壁间产生大量贯通状大气孔,气孔孔径增大㊂图7(b)㊁(c)为经1260ħ烧后试样断口处的SEM照片,当烧成温度上升到1260ħ时,板状钙长石尺寸明显增大且数量增多㊂此外,图8给出了烧成温度为1260ħ时试样孔壁位置的SEM照片,并采用Factsage计算了1260ħ烧后试样玻璃相的化学组成,如表2所示㊂可以看出,当烧成温度为1260ħ时,玻璃相成分主要为SiO2㊁Al2O3和CaO,且均匀地分布在竖条状的钙长石晶粒之间㊂第3期陈㊀曦等:烧成温度对钙长石隔热耐火材料显微结构与性能的影响1127㊀图6㊀经1210ħ烧后试样的SEM 照片Fig.6㊀SEM images of samples sintered at 1210ħ图7㊀经1260ħ烧后试样的SEM 照片Fig.7㊀SEM images of samples sintered at 1260ħ图8㊀经1260ħ烧后试样抛光后孔壁内部的SEM 照片Fig.8㊀SEM images of pore wall of polished samples sintered at 1260ħ图9给出了经1310ħ烧后试样的抛光面与断口处的SEM 照片㊂图9(a)为1310ħ烧后试样抛光面的SEM 照片,当烧成温度为1310ħ时,试样的收缩程度大幅上升,孔壁厚度增加,孔壁内小气孔和孔壁间大气孔的孔径明显减小㊂图9(b)㊁(c)为经1310ħ烧后试样断口处的SEM 照片,当烧成温度为1310ħ时,板状钙长石完全析出且晶粒发育完全,晶粒较为致密㊂为进一步研究烧后试样的气孔特性,图10给出了各试样的孔径分布和累积分布㊂由图可知,烧后试样的孔径均呈双峰分布㊂孔壁内的小气孔介于3~92μm,孔壁间的大气孔介于100~1000μm㊂随着烧成温度由1110ħ升高至1260ħ,小孔峰逐渐下降且向右移动,大孔峰右移㊂当烧成温度进一步升高至1310ħ时,小孔峰升高且左移,大孔峰左移㊂当烧成温度由1110ħ上升到1260ħ时,气孔的中位径由221μm 上升到474μm,继续升高烧成温度到1310ħ,中位径显著降低到197μm㊂表2㊀经1260ħ烧后试样玻璃相的化学组成Table 2㊀Chemical composition of glass phase of sample sintered at 1260ħComposition Al 2O 3SiO 2Fe 2O 3CaO MgO K 2O Na 2O TiO 2Mass fraction /%27.6640.170.7420.160.590.27 6.32 4.092.3㊀物理性能图11为经不同温度烧后试样的总气孔率和体积密度㊂当烧成温度从1110ħ升高至1260ħ时,总气孔率从85.7%降低至82.6%,体积密度从0.43g /cm 3增大至0.53g /cm 3㊂当烧成温度进一步升高到1310ħ1128㊀耐火材料硅酸盐通报㊀㊀㊀㊀㊀㊀第43卷时,总气孔率显著降低至76.7%,体积密度增大至0.71g /cm 3㊂图9㊀经1310ħ烧后试样的SEM 照片Fig.9㊀SEM images of samples sintered at 1310ħ图10㊀经不同温度烧后试样的孔径分布曲线和累积分布曲线Fig.10㊀Pore size distribution and cumulative distribution curves of samples sintered at different temperatures 图12为经不同温度烧后试样的抗折强度与耐压强度㊂当烧成温度从1110ħ升高至1260ħ时,抗折强度从0.38MPa 增大至1.15MPa,耐压强度从0.86MPa 增大至1.99MPa㊂当烧成温度进一步升高到1310ħ时,抗折强度显著增大至2.54MPa,耐压强度增大至4.94MPa㊂图11㊀经不同温度烧后试样的总气孔率和体积密度Fig.11㊀Total porosity and bulk density of samples sintered at differenttemperatures 图12㊀经不同温度烧后试样的抗折强度与耐压强度Fig.12㊀Flexural strength and compressive strength of samples sintered at different temperatures图13为1260ħ烧后试样在不同温度下的导热系数,随着测试温度由350ħ升高到1000ħ,试样的导热系数由0.085W /(m㊃K)上升到0.112W /(m㊃K),其中800ħ时的导热系数为0.101W /(m㊃K)㊂表3第3期陈㊀曦等:烧成温度对钙长石隔热耐火材料显微结构与性能的影响1129㊀给出了其他钙长石隔热耐火材料与本研究的导热系数比较,从表中可以看出,相比其他钙长石隔热耐火材料,尽管本研究试样体积密度较高,但其导热系数较低㊂图13㊀试样经1260ħ烧后在不同温度下的导热系数Fig.13㊀Thermal conductivity of sample sintered at 1260ħat different test temperatures表3㊀其他钙长石隔热耐火材料的导热系数与本研究的比较Table 3㊀Comparison of thermal conductivity of other anorthite insulation refractories with this studyProduct Bulk density /(g㊃cm -3)Thermal conductivity(800ħ)/(W㊃m -1㊃K -1)Commercially available product [21]0.4~0.650.19Anorthite lightweight brick Jm23[22]0.480.17Liu [22]0.450.16Ni [14]0.470.17Priogov [23]0.450.16This study(1260ħ)0.530.102.4㊀分析讨论由上述结果可知,烧成温度对钙长石隔热耐火材料显微结构和性能有重要影响,当烧成温度为1260ħ时,材料时兼具高强度和低导热系数㊂影响钙长石隔热耐火材料显微结构与性能的主要因素包括成型与烧成过程中的水泥水化㊁钙长石形成以及气孔结构演变㊂当温度大于30ħ时,生坯中的水泥会发生式(1)㊁(2)所示的水化反应,水泥的水化反应会放出大量热,对浇注后的坯体起到很大程度的助凝作用,使得湿坯体能够快速凝固硬化,从而阻止小气孔合并成为大气孔㊂CaAl 2O 4+n H 2O ңC 3AH 6+AH 3(1)CaAl 4O 7+n H 2O ңC 3AH 6+AH 3(2)图14㊀不同温度下试样中液相的含量和黏度Fig.14㊀Liquid phase content and liquid phase viscosity of samples at different temperatures图14为不同温度下试样中液相的含量和黏度㊂在烧成过程中,随着温度的升高,水泥的水化产物进一步分解成CaAl 2O 4(CA)和CaAl 4O 7(CA 2)[24],黏土脱去结合水,生成偏高岭石[25-26],形成具有微孔的黏土假象㊂当烧成温度升高至1110~1210ħ时,试样中高温液相含量增大至2.49%~3.42%,黏度减小至6.54ˑ104~1.95ˑ103Pa㊃s,来自水泥颗粒中的CA㊁CA 2和黏土假象中的SiO 2会发生反应生成钙铝黄长石(如式(3)㊁(4)所示);随后钙铝黄长石与液相中的Al 2O 3和SiO 2反应生成钙长石(如式(5)所示)㊂液相含量的增加以及黏度的降低一方面增大了钙长石的生成速率;另一方面,促进了颗粒间的反应,孔壁间的原料颗粒逐渐减少(图5㊁图6),孔壁内的小气孔向孔1130㊀耐火材料硅酸盐通报㊀㊀㊀㊀㊀㊀第43卷壁间大气孔中迁移,大气孔孔径增大,孔壁致密化程度逐渐增大,孔壁厚度减小,总气孔率降低,强度增加㊂2CaAl2O4+SiO2ңCa2Al2SiO7+Al2O3(3)2CaAl4O7+SiO2ңCa2Al2SiO7+3Al2O3(4)Ca2Al2SiO7+Al2O3+3SiO2ң2CaAl2Si2O8(5)当烧成温度升高至1260ħ时,反应烧结的致密化进程加快㊂此时,试样中高温液相含量增大至5.92%,高温液相含量的进一步增大促进了液相对颗粒的润湿和扩散行为,增加了液相与颗粒之间的接触面积,增大了钙铝黄长石向液相中的溶解速率,促进了钙长石晶粒的析出(见式(5))㊂因此,试样孔壁间的原料颗粒全部反应生成钙长石,孔壁内的小气孔向孔壁间大气孔中迁移的速率增大,大气孔孔径增加,且孔壁致密化程度增大,孔壁厚度显著减小,总气孔率降低,但强度进一步增大㊂继续升高温度至1310ħ时,液相含量急剧升高至12.23%,此时在反应烧结和液相烧结的共同作用下,虽然烧结致密化程度大幅增加,但试样的线收缩率急剧增大,孔壁厚度显著增加,且试样的收缩速率远远大于孔壁内小气孔向孔壁间大气孔的迁移速率,这就使得试样孔壁间气孔孔径大幅减小,气孔率显著降低,表现出增强的力学性能㊂综合比较各温度烧后试样的物理性能及显微结构,当烧成温度为1260ħ时,试样具有最优性能,均匀且较小的孔径使得试样具有较低的导热系数,此时烧后试样的物相组成仅为钙长石㊂表4给出了其他钙长石隔热耐火材料与本研究制品在不同服役温度下玻璃相的黏度对比㊂由表可知,对比于其他钙长石隔热耐火材料制品,本研究制品的玻璃相含量较低,在850~1050ħ的使用温度下,本制品玻璃相黏度略高于其他制品,较少含量和较高黏度的玻璃相均匀分布在钙长石之间[27-29],形成钙长石-玻璃相结构,在一定程度上降低了钙长石隔热耐火材料的导热系数㊂表4㊀其他钙长石隔热耐火材料与本研究制品在不同服役温度下玻璃相的黏度对比Table4㊀Comparison of viscosity of glass phase between other anorthite insulation refractories andthis research product at different service temperaturesProduct Viscosity/(Pa㊃s)850ħ900ħ950ħ1000ħ1050ħThis study(Glass phase content:5.92%) 4.40ˑ108 3.99ˑ106 4.93ˑ1057.79ˑ104 1.50ˑ104 Gu[13](Glass phase content:12.17%) 6.89ˑ1067.10ˑ105 1.02ˑ105 1.92ˑ104 4.46ˑ103 Anorthite lightweight brick Jm23[22](Glass phase content:9.67%) 1.74ˑ107 2.01ˑ106 3.10ˑ105 5.95ˑ104 1.36ˑ1043㊀结㊀论1)当烧成温度从1110ħ升高至1210ħ时,原料颗粒之间的反应加剧,优先生成钙铝黄长石,同时钙铝黄长石反应生成钙长石的速度逐渐加快;当烧成温度升高至1260ħ时,孔壁间的原料颗粒反应完全,钙铝黄长石全部反应生成钙长石;进一步升高温度至1310ħ时,液相含量急剧增多,钙长石部分溶解进入液相中,生成少量的钙铝榴石和红铝透辉石㊂2)当烧成温度为从1110ħ升高至1260ħ时,孔壁间的原料颗粒逐渐减少,孔壁内小气孔向孔壁间大气孔迁移,孔壁致密化程度逐渐增大,总气孔率降低,强度提高;继续升高温度至1310ħ,液相含量急剧升高,在反应烧结和液相烧结的共同作用下,烧结致密化程度大幅增加,试样的收缩速率远远大于孔壁内小气孔向孔壁间大气孔的迁移速率,试样孔径减小且气孔率降低㊂3)当烧成温度为1260ħ时,钙长石隔热耐火材料具有最优异的综合性能,总气孔率为82.6%,中位孔径为474μm,抗折强度为1.15MPa,耐压强度为1.99MPa,800ħ时的导热系数为0.101W/(m㊃K),与市售钙长石隔热耐火材料相比,导热系数降低了46.8%㊂参考文献[1]㊀WANG H L,LI Y B,YIN B,et al.Synthesis and application evaluation in lithium battery furnace of mullite insulating refractory bricks fromtailings[J].International Journal of Applied Ceramic Technology,2023,20(5):3237-3245.[2]㊀WANG S Y,QI X,QI D B,et al.Study on the properties of periclase-forsterite lightweight heat-insulating refractories for ladle permanent㊀第3期陈㊀曦等:烧成温度对钙长石隔热耐火材料显微结构与性能的影响1131 layer[J].Ceramics International,2022,48(14):20275-20284.[3]㊀TORMAN K M,ERDO㊅GAN G,YAVAŞA,et al.Fabrication of porous anorthite ceramics using eggshell waste as a calcium source and expandedpolystyrene granules[J].Politeknik Dergisi,2022,25(3):1235-1241.[4]㊀SEMENOVYKH M A,SKRIPNIKOVA N K,SHEKHOVTSOV V V.High-temperature synthesis of anorthite phase in glass ceramicmaterial[C]//Physical Mesomechanics of Condensed Matter:physical principles of multiscale structure formation and the mechanisms of nonlinear behavior.AIP Conference Proceedings.Tomsk,Russia:AIP Publishing,2023.[5]㊀郭会师,杨佳麟,李文凤,等.铝硅系原料种类对钙长石质隔热耐火材料性能的影响[J].耐火材料,2023,57(4):286-290.GUO H S,YANG J L,LI W F,et al.Effects of Al2O3-SiO2raw material types on properties of anorthite based insulation refractories[J].Refractories,2023,57(4):286-290(in Chinese).[6]㊀ASHRAF E,SHOKHIDA K,ABDULAZIZ K.Recycling industrial waste into high-performance ceramics[J].Universum,2023,3:22-26.[7]㊀SUTCU M,AKKURT S,BAYRAM A,et al.Production of anorthite refractory insulating firebrick from mixtures of clay and recycled paper wastewith sawdust addition[J].Ceramics International,2012,38(2):1033-1041.[8]㊀付梦丽,李翠伟,武令豪,等.环境友好的泡沫注凝法制备钙长石多孔陶瓷[J].硅酸盐学报,2022,50(3):723-728.FU M L,LI C W,WU L H,et al.Fabrication of porous anorthite ceramics by environmental-friendly foam gel-casting method[J].Journal of the Chinese Ceramic Society,2022,50(3):723-728(in Chinese).[9]㊀刘静静.燃尽物法制备氧化铝隔热耐火材料孔结构与性能相关性研究[D].武汉:武汉科技大学,2016:11-14.LIU J J.Study on the correlation between pore structure and properties of alumina thermal insulation refractory prepared by burnout method[D].Wuhan:Wuhan University of Science and Technology,2016:11-14(in Chinese).[10]㊀LI H A,XU C S,CHEN J T,et al.Effect of different particle sizes and pore-making agent ratio on properties of SiC porous ceramics[J].Journalof Physics:Conference Series,2020,1622(1):012042.[11]㊀LI C W,HAN Y,WU L H,et al.Synthesis and growth of anorthite crystal during in situ preparation of porous anorthite ceramics by foam-gelcasting[J].International Journal of Applied Ceramic Technology,2017,14(5):957-962.[12]㊀韩㊀耀.钙长石基多孔陶瓷的结构设计㊁制备及性能研究[D].北京:北京交通大学,2014:31-37.HAN Y.Structural design,preparation and properties of anorthite-based porous ceramics[D].Beijing:Beijing Jiaotong University,2014:31-37 (in Chinese).[13]㊀顾幸勇,马光华.钙长石质轻质隔热材料研制[J].陶瓷学报,1998,19(3):144-148.GU X Y,MA G H.Studying on the light anorthite type of heat-isolated material[J].Journal of Ceramics,1998,19(3):144-148(in Chinese).[14]㊀倪㊀文,刘凤梅,李翠伟.利用天然原料合成钙长石轻质耐火砖的研究[J].地质找矿论丛,1998,13(2):1-9.NI W,LIU F M,LI C W.A study of anorthite light refractory brick s synthesis by using natural minerals[J].Conributions to Geology and Mineral Resources Research,1998,13(2):1-9(in Chinese).[15]㊀耿浩洋,潘志华.化学发泡法制备钙长石系轻质隔热砖坯体[J].硅酸盐通报,2013,32(5):814-818.GENG H Y,PAN Z H.Preparation of anorthite lightweight thermal insulation raw bricks by means of chemically foaming process[J].Bulletin of the Chinese Ceramic Society,2013,32(5):814-818(in Chinese).[16]㊀CHANDRASEKHAR S,RAGHAVAN P,SEBASTIAN G,et al.Brightness improvement studies on kaolin based zeolite4A[J].Applied ClayScience,1997,12(3):221-231.[17]㊀刘㊀强,潘志华,李庆彬,等.钙长石系轻质隔热砖的制备及钙长石形成过程[J].硅酸盐通报,2010,29(6):1269-1274.LIU Q,PAN Z H,LI Q B,et al.Preparation of anorthite lightweight thermal insulating brick and the formation process of anorthite[J].Bulletin of the Chinese Ceramic Society,2010,29(6):1269-1274(in Chinese).[18]㊀李㊀楠,鄢㊀文,李媛媛.莫来石-高硅氧玻璃复合材料及其应用[C]//第六届国际耐火材料会议论文集,2012:30-33.LI N,YAN W,LI Y Y.Mullite-high silica glass composites and their applications[C]//Proceedings of the6th International Conference on Refractories,2012:30-33(in Chinese).[19]㊀李媛媛,李㊀楠,鄢㊀文,等.结合剂对莫来石-高硅氧玻璃复合材料轻骨料浇注料性能的影响[C]//第六届国际耐火材料会议论文集,2012:60-62.LI Y Y,LI N,YAN W,et al.Effect of bonding agent on castable properties of lightweight aggregate of mullite-high silica glass composite[C]// Proceedings of the6th International Conference on Refractories,2012:60-62(in Chinese).[20]㊀MIAO Z,LI N,YAN W.Effect of sintering temperature on the phase composition and microstructure of anorthite-mullite-corundum porousceramics[J].Ceramics International,2014,40(10):15795-15799.[21]㊀孙祥云,周季婻,于祥旭,等.钙长石质轻质隔热耐火材料及其制备方法:CN101781120A[P].2010-07-21.SUN X Y,ZHOU J N,YU X X,et al.Calcareous lightweight thermal insulation refractory and its preparation method:CN101781120A[P].2010-07-21(in Chinese).[22]㊀刘㊀杰.新型钙长石轻质耐火材料的制备工艺研究[D].景德镇:景德镇陶瓷学院,2010:37-38.1132㊀耐火材料硅酸盐通报㊀㊀㊀㊀㊀㊀第43卷LIU J.Study on preparation technology of new anorthite lightweight refractory[D].Jingdezhen:Jingdezhen Ceramic Institute,2010:37-38(in Chinese).[23]㊀PIROGOV A A,RAKINA V P,MIRAK YAN M M,et al.Anorthite insulating refractory[J].Refractories,1970,11(5/6):303-307.[24]㊀杨守磊,肖国庆,丁冬海,等.CaO-Al-Al2O3-CaCO3-O2体系燃烧合成铝酸钙热力学研究[J].硅酸盐学报,2016,44(6):908-913.YANG S L,XIAO G Q,DING D H,et al.Thermodynamic analysis of combustion synthesis of calcium aluminate in CaO-Al-Al2O3-CaCO3-O2 system[J].Journal of the Chinese Ceramic Society,2016,44(6):908-913(in Chinese).[25]㊀齐仕杰.利用废弃混凝土和废弃粘土砖制备蒸压砖[D].大连:大连理工大学,2021:27-32.QI S J.Preparation of autoclaved brick from waste concrete and waste clay brick[D].Dalian:Dalian University of Technology,2021:27-32(in Chinese).[26]㊀李亚伟,李㊀楠,李文忠,等.碳热还原粘土合成Al2O3/SiC复相陶瓷粉末[J].中国陶瓷,2000,36(3):13-15+35.LI Y W,LI N,LI W Z,et al.Synthesis of Al2O3/SiC composite in carbothermal reduction of clay mineral[J].China Ceramics,2000,36(3): 13-15+35(in Chinese).[27]㊀董伟霞,包启富,顾幸勇,等.工艺制备对钙长石/莫来石复合材料力学性能的影响[J].陶瓷学报,2011,32(2):216-220.DONG W X,BAO Q F,GU X Y,et al.Effect of preparation process on mechanical properties of anorthite/mullite composites[J].Journal of Ceramics,2011,32(2):216-220(in Chinese).[28]㊀李㊀帅,张㊀快,李运刚.钙长石材料的研究现状综述[J].中国陶瓷,2022,58(4):16-21.LI S,ZHANG K,LI Y G.Review on research status of anorthite materials[J].China Ceramics,2022,58(4):16-21(in Chinese). [29]㊀SHEN C,HUANG Y L,ZHANG Y N,et al.Preparation and properties of anorthite-based ceramics by using metallurgical solid waste and flyash[J].International Journal of Applied Ceramic Technology,2023,20(4):2301-2308.。
Microstructure and mechanical properties of twinned
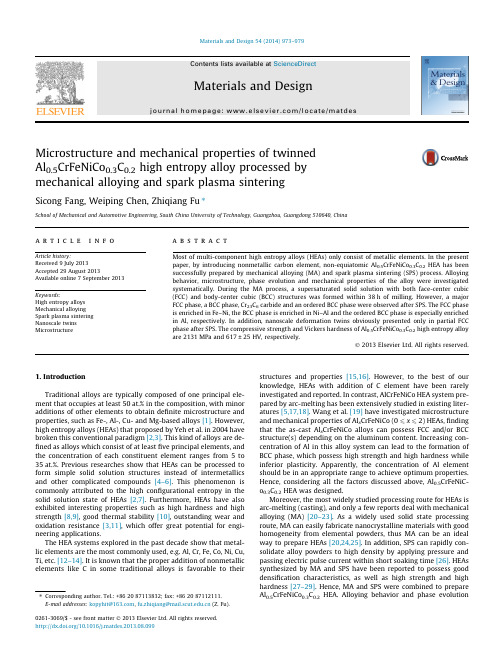
Microstructure and mechanical properties of twinned Al 0.5CrFeNiCo 0.3C 0.2high entropy alloy processed by mechanical alloying and spark plasmasinteringSicong Fang,Weiping Chen,Zhiqiang Fu ⇑School of Mechanical and Automotive Engineering,South China University of Technology,Guangzhou,Guangdong 510640,Chinaa r t i c l e i n f o Article history:Received 9July 2013Accepted 29August 2013Available online 7September 2013Keywords:High entropy alloys Mechanical alloying Spark plasma sintering Nanoscale twins Microstructurea b s t r a c tMost of multi-component high entropy alloys (HEAs)only consist of metallic elements.In the present paper,by introducing nonmetallic carbon element,non-equiatomic Al 0.5CrFeNiCo 0.3C 0.2HEA has been successfully prepared by mechanical alloying (MA)and spark plasma sintering (SPS)process.Alloying behavior,microstructure,phase evolution and mechanical properties of the alloy were investigated systematically.During the MA process,a supersaturated solid solution with both face-center cubic (FCC)and body-center cubic (BCC)structures was formed within 38h of milling.However,a major FCC phase,a BCC phase,Cr 23C 6carbide and an ordered BCC phase were observed after SPS.The FCC phase is enriched in Fe–Ni,the BCC phase is enriched in Ni–Al and the ordered BCC phase is especially enriched in Al,respectively.In addition,nanoscale deformation twins obviously presented only in partial FCC phase after SPS.The compressive strength and Vickers hardness of Al 0.5CrFeNiCo 0.3C 0.2high entropy alloy are 2131MPa and 617±25HV,respectively.Ó2013Elsevier Ltd.All rights reserved.1.IntroductionTraditional alloys are typically composed of one principal ele-ment that occupies at least 50at.%in the composition,with minor additions of other elements to obtain definite microstructure and properties,such as Fe-,Al-,Cu-and Mg-based alloys [1].However,high entropy alloys (HEAs)that proposed by Yeh et al.in 2004have broken this conventional paradigm [2,3].This kind of alloys are de-fined as alloys which consist of at least five principal elements,and the concentration of each constituent element ranges from 5to 35at.%.Previous researches show that HEAs can be processed to form simple solid solution structures instead of intermetallics and other complicated compounds [4–6].This phenomenon is commonly attributed to the high configurational entropy in the solid solution state of HEAs [2,7].Furthermore,HEAs have also exhibited interesting properties such as high hardness and high strength [8,9],good thermal stability [10],outstanding wear and oxidation resistance [3,11],which offer great potential for engi-neering applications.The HEA systems explored in the past decade show that metal-lic elements are the most commonly used,e.g.Al,Cr,Fe,Co,Ni,Cu,Ti,etc.[12–14].It is known that the proper addition of nonmetallic elements like C in some traditional alloys is favorable to theirstructures and properties [15,16].However,to the best of our knowledge,HEAs with addition of C element have been rarely investigated and reported.In contrast,AlCrFeNiCo HEA system pre-pared by arc-melting has been extensively studied in existing liter-atures [5,17,18].Wang et al.[19]have investigated microstructure and mechanical properties of Al x CrFeNiCo (06x 62)HEAs,finding that the as-cast Al x CrFeNiCo alloys can possess FCC and/or BCC structure(s)depending on the aluminum content.Increasing con-centration of Al in this alloy system can lead to the formation of BCC phase,which possess high strength and high hardness while inferior plasticity.Apparently,the concentration of Al element should be in an appropriate range to achieve optimum properties.Hence,considering all the factors discussed above,Al 0.5CrFeNiC-o 0.3C 0.2HEA was designed.Moreover,the most widely studied processing route for HEAs is arc-melting (casting),and only a few reports deal with mechanical alloying (MA)[20–23].As a widely used solid state processing route,MA can easily fabricate nanocrystalline materials with good homogeneity from elemental powders,thus MA can be an ideal way to prepare HEAs [20,24,25].In addition,SPS can rapidly con-solidate alloy powders to high density by applying pressure and passing electric pulse current within short soaking time [26].HEAs synthesized by MA and SPS have been reported to possess good densification characteristics,as well as high strength and high hardness [27–29].Hence,MA and SPS were combined to prepare Al 0.5CrFeNiCo 0.3C 0.2HEA.Alloying behavior and phase evolution0261-3069/$-see front matter Ó2013Elsevier Ltd.All rights reserved./10.1016/j.matdes.2013.08.099Corresponding author.Tel.:+862087113832;fax:+862087112111.E-mail addresses:kopyhit@ ,fu.zhiqiang@ (Z.Fu).during milling and consolidation processesied.Microstructure and mechanical properties ofwere investigated.2.Experiment proceduresHEA with a nominal composition of Al0.5pressed in molar ratio)was prepared by drythen by wet milling for4h in ethanol.Al,Cr,Fe,elemental powders with purity higher than99.9size of645l m(325mesh)were mechanicallywas carried out in a high energy planetaryPlanetary Ball Mill)at300rpm with a ball toof10:1under argon atmosphere.Highvials and tungsten carbide balls(10mm inthe milling media.In order to confirm the alloymilling,powder samples were taken out after27,38,42h respectively.The42h ball milledsubsequently sintered by Dr.Sinter Model SPS-825Spark PlasmaSintering System(Sumitomo Coal Mining Co.Ltd.,Japan)at 1273K for8min at a pressure of30MPa under vacuum(residual cell pressure<8Pa).The samples were heated to873K within 4min,while from873K to1173K and from1173K to1273K, heating rates of75K minÀ1and50K minÀ1were used, respectively.The milled powders and the bulk alloy after sintering were ana-lyzed by a Bruker D8ADVANCE X-ray diffractometer(XRD)with a Cu K a radiation.The microstructure of the alloy was revealed by etching in aqua regia and observed using scanning electron micros-copy(SEM,Zeiss Supra40,Carl Zeiss NTS GmbH,Germany).Thin-foil specimens were prepared by mechanical thinning followed by ion milling at room temperature and were analyzed by a transmis-sion electron microscopy(200kV TEM,JEM-2100,JEOL,Japan) with selected area electron diffraction(SAED)analysis.According to GB/T7314-2005[30],the room-temperature compressive prop-erties of the cylindrical samples(U3mmÂ4.5mm in size)were measured with an Instron5500testing system at a strain rate of 1Â10À3sÀ1.Three compression tests were performed to obtain average value.Hardness measurement was conducted using a Dig-ital Micro Hardness Tester HVS-1000Vickers hardness instrument under a load of300gf.The reported hardness value is an average of at least10measurements.3.Results and discussion3.1.XRD analysis and microstructure during MAFig.1shows the XRD patterns of Al0.5CrFeNiCo0.3C0.2HEA pow-ders prepared under different milling durations.It can be seen that diffraction patterns of all alloy elements are included in the initial blending powder.As the milling time increases,drastic decrement of diffraction intensity is observed after6h of milling.The peaks of Al,Co and C elements dissolve most rapidly.According to Chen et al.[31],the alloying sequence for the Cu0.5NiAlCoCrFeTiMo alloy system correlates best with the melting point of the component elements.Thus rapid dissolution of both Al and Co could be asso-ciated with their lower melting point than other elements.In con-trast,the early disappearance of diffraction peak of C may result from its smallest atomic fraction in the alloy.Many diffraction peaks can hardly be seen when the milling time reaches up to 27h.The complete disappearance of all the elemental peaks and the formation of solid solution are founded within38h of milling. Predominant peaks corresponding to an face-centered cubic(FCC) and a body-centered cubic(BCC)crystal structures are respectively observed.Subsequently,the powder was subjected to wet ball milling in ethanol for4h with the aim to obtainfine metallic pow-der for being conductive to sintering.As the milling time extends to42h,the diffraction peaks exhibit no change except for a minor broadening and a significant increment of diffraction intensity.The crystal size and lattice strain of the BCC and FCC phases with different milling time have been calculated from the X-ray peak broadening using Scherrer’s formula after deducting the instrumental contribution.The calculated results are listed in Table1.The crystalline sizes of both BCC and FCC phases after 42h of milling are slightly refined compared with38h of milling, while the lattice strain of these two phases increase as the milling time prolongs.The crystallite refinement can be attributed to the circulation of crushment and agglomeration during the MA process.Reasons for the increment of lattice strain include size mismatch effect between the constituent elements,increasing grain boundary fraction and high dislocation density imparted by MA[21].The crystallite refinement and high lattice strain may account for the above-mentioned intensity increment and peak broadening in the diffraction.The SEM images of the Al0.5CrFeNiCo0.3C0.2HEA powders of dif-ferent milling time are shown in Fig.2.The primitive powder shows a granular size of less than40l m.In the early period of MA(as shown in Fig.2(b)),the particles cold weld together to form even larger particles than that of primitive powder.Subsequently, when the milling time reaches15h(Fig.2(c)),most of the cold welded agglomerations are crushed down to smaller particles. The27h milled alloy powder reveals an average particle size of approximately5l m as shown in Fig.2(d),and the particles cold weld again when the milling time reaches38h(Fig.2(e)).This cir-culation of crushing and cold welding induced by the ball mill gradually reduces the crystalline size and facilitates the diffusion and alloying among different elements.The38h milled alloy pow-der is subsequently wet ball milled for4h with alcohol as the mill-ing media.It can be seen from Fig.2(f)that the elliptoid particles are fractured,exhibiting a lamellar structure.The particle size for thefinal powder is much smaller than that of38h milled powder.1.XRD patterns of Al0.5CrFeNiCo0.3C0.2HEA powders with different milling time.Table1The crystalline size and lattice strain of Al0.5CrFeNiCo0.3C0.2HEA during MA.Milling time(h)Crystalline size(nm)Lattice strain(%)BCC FCC BCC FCC 3812130.710.734211120.760.77974S.3.2.Phase evolution and microstructure after SPSFig.3illustrates the XRD pattern of Al0.5CrFeNiCo 0.3C 0.2HEA detailed analysis of the XRD pattern suggests a BCC phase,a Cr 23C 6carbide phase and an visible.Except the ordered BCC phase,the other three phases are calculated to be (BCC),10.652Å(Cr 23C 6),respectively.After the FCC phase reduces from 0.77%after MA phase from 0.76%to 0.27%,which confirmsthe nearly annihilation of defects introduced during MA after sin-tering.As hereinbefore mentioned,the main phases of Al 0.5CrFe-NiCo 0.3C 0.2HEA powder after 42h of milling are the BCC and FCC solid-solution phases,demonstrating that densification at 1273K for 8min has resulted in phase evolution.The MA process could in-duce large strain and defects which might lead to the extension of solubility,thus the milled powders are generally in a non-equilib-rium state.It is certain that a reordering process happened during SPS,leading to the metastable supersaturated solid solutions of MA to more stable phases.This reordering can be correlated with the above-mentioned annihilation of defects introduced by severe plastic deformation during MA.Furthermore,the huge pulsed elec-tric current during the SPS process can also facilitate the phase evolution.Fig.4shows the SEM micrographs of bulk Al 0.5CrFeNiCo 0.3C 0.2HEA consolidated by SPS.Few porosities can be seen in low magni-fied image (Fig.4(a)).Actually,relative density of the sintered Al 0.5-CrFeNiCo 0.3C 0.2HEA sample,which is calculated with respect to theoretical density,reaches up to 99.6%.Two distinctive areas are visible in Fig.4(a),viz.,irregular bulk areas and irregular pot hole areas.The irregular bulk areas are most likely to be the main phase because they account for a higher volume fraction.While the pot hole areas can be consist of phases which are removed by corrosive agent.High magnified image (Fig.4(b))shows that some nanoscale black spots are dispersed in irregular pot hole areas.Most of these spots might be numerous nanoscale phases,and a small minority of spots might be ultrafine porosities.In summary,bulk Al 0.5CrFe-NiCo 0.3C 0.2HEA might consist of at least three types of phases.The TEM bright field image and corresponding selected area electron diffraction (SAED)patterns of Al 0.5CrFeNiCo 0.3C 0.2HEAAl 0.5CrFeNiCo 0.3C 0.2HEA powders with different milling time.(a)0h,(b)6h,(c)15h,(d)27h,(e)38h and (f)Fig.3.XRD pattern of Al 0.5CrFeNiCo 0.3C 0.2HEA after SPS.after SPS are shown in Fig.5.In order to confirm the phase compo-sition of Al 0.5CrFeNiCo 0.3C 0.2HEA,EDS/TEM method was used to measure chemical compositions (in at.%)of the phases in Fig.5(a)(regions marked A–Y).Results of the chemical composi-tion analysis are listed in Table 2.It is noticed that all the regions can be classified as four different phases.Crystal structures of these phases are measured by their corresponding SAED patterns as shown from Fig.5(b–e).As a result,these four phases are measured to be an FCC phase,a BCC phase,Cr 23C 6carbide and an ordered BCC phase,respectively.It is worth mentioning that diffraction pattern of region C exhibits a FCC twin structure of the FCC phase.Clear microstructures and corresponding SAED patterns of twins will be illustrated hereinafter.As shown in Table 2,the FCC phase isdepleted in Al,Cr compared with the nominal CrFeNiCo 0.3C 0.2HEA.The BCC phase is Ni-rich,Fe-depleted and C-depleted,while the or-greatly rich in Al.The lattice parameters of measured by SAED are 3.700Å(FCC),Å(Cr 23C 6)and 4.320Å(Ordered BCC),Cr 23C 6phase also presents Fe,Ni,Co and Al elements,especially Fe shows a high concentration (12.7±0.4at.%).In conclusion,the bulk Al 0.5CrFeNiCo 0.3C 0.2HEA exhibits a Fe–Ni-rich FCC phase,a Ni ÀAl-rich BCC phase,a Cr 23C 6carbide phase and a Al-rich ordered BCC phase,which is in accordance with the XRD result (Fig.3).It is noticeable that the grain size of these phases shows a wide distri-bution ranging from several hundred nanometers to 1l m.How-ever,the Cr 23C 6and the ordered BCC phases are much finer than the FCC and the BCC phases.It is a reasonable explanation that the Cr 23C 6and the ordered BCC phases are formed in a phase evo-lution and reordering process during SPS.The formation of these four phases is complicated.According to the Gibbs phase rules,the number of equilibrium phases (p )is p =n +1for the alloy that contains n elements.Since phase formation not in equilibrium conditions,the number p >n +1.However,the Al 0.5CrFeNiCo 0.3C 0.2four types of phases,showing much fewer Yeh et al.[2]has proposed that it is possible solutions when HEAs contain five or more 4.SEM micrographs of Al 0.5CrFeNiCo 0.3C 0.2HEA after SPS.(a)low-magnified image,and (b)high-magnified image.corresponding SAED patterns of bulk Al 0.5CrFeNiCo 0.3C 0.2HEA after SPS.(a)bright field image,(b)SAED pattern of FCC [011]1]zone axis (Region D),(d)SAED pattern of Cr 23C 6[112]zone axis (Region B),and (e)SAED pattern of ordered BCC [012]Table 2Chemical composition (in at.%)analysis results of the phases by EDS/TEM.RegionsPhases Cr Fe Ni Al Co C A,C,F,G,I,J,K,M,N,O,P,T,V,W,X,Y FCC 21.8±0.931.8±1.229±1.1 4.4±0.68.5±0.8 4.5±0.5D,E,Q,R BCC 19.7±1.218.6±2.032.8±1.217.5±1.87.2±0.5 4.2±0.4B,L Cr 23C 663.5±2.112.7±0.4 2.9±0.4 1.8±0.3 2.6±0.316.5±1.6H,S,UOrdered BCC 1.6±0.5 2.4±0.42±0.486.8±1.7 1.2±0.46±1.0Nominal composition–25252512.57.55are randomly distributed in the crystal lattice,though the alloy exhibits high mixing entropy.It reveals that high mixing entropy is insufficient to dominate the formation of phases in HEAs sys-tems.Zhang et al.[32]related the formation of simple solid solu-tion to the mixing enthalpy (D H mix )and atomic size difference (d ).Subsequently,Yang and Zhang [33]proposed a solid-solution formation rule for multi-component HEAs based on the calculation of most of reported HEAs.According to Yang et al.,two parameters can be used to estimate the phase formation behavior of HEAs:O is defined as a parameter of the entropy of mixing timing the average melting temperature of the elements over the enthalpy of mixing,d is defined as the mean square deviation of the atomic size of ele-ments.It is proposed that HEAs form simple crystal structures when O P 1.1and d 66.6%.These two parameters are defined by Eqs.(1)and (2),respectively.X ¼T m D S mix j D H mix jð1Þd ¼ffiffiffiffiffiffiffiffiffiffiffiffiffiffiffiffiffiffiffiffiffiffiffiffiffiffiffiffiffiffiffiffiffiffiffiffiffiffiX n i ¼1c i ð1Àr i = r Þ2q ð2Þwhere c i is the atomic percentage of the i th component, r ¼Pn i ¼1c i r i is the average atomic radius and r i is the atomic radius of the i th component.T m ,D S mix and D H mix are calculated as follows:T m ¼Xn i ¼1c i ðT m Þið3ÞHere,(T m )i is the melting point of the i th component of alloy.D S mix¼ÀR Xn i ¼1ðc i ln c i Þð4Þwhere c i is the mole percent of component,P ni ¼1c i¼1,and R(=8.314JK À1mol À1)is gas constant.D H mix ¼X n i ¼1;i –jX ij c i c j ð5Þwhere X ij ¼4D H mix ijis the regular solution interaction parameter between the i th and j th elements,c i or c j is the atomic percentage of the i th or j th component,and D H mix ijis the enthalpy of mixing of binary liquid alloys.Table 3presents the value of mixing enthal-py of atom-pairs of Al 0.5CrFeNiCo 0.3C 0.2HEA.The calculated results of O and d for the alloy are 1.41and 10.01%,respectively.It is obvi-ous that O well matches while d breaks the solid-solution formation rules for the multi-component high entropy alloys proposed by Yang et al.This phenomenon indicates that the formation of Cr 23C 6carbide and ordered BCC phase is reasonable in this alloy sys-tem and it well conforms to Yang’s research.Firstly,it is obvious that the value of O shows the relative predominance of T m D S mix and D H mix .Since O >1is obtained,T m D S mix should be the predomi-nant part of the free energy.It is known that mixing entropy indi-cates the tendency for the formation of random solution while enthalpy of mixing in any system indicates the tendency for order-ing or clustering,thus the formation of solid-solution phases ought to be much easier than the formation of intermetallic compoundsand other ordered phases in the Al 0.5CrFeNiCo 0.3C 0.2HEA.Obviously,the formation of FCC solid-solution phase as the main phase of Al 0.5-CrFeNiCo 0.3C 0.2HEA is mainly correlated with the alloy’s high mix-ing entropy.Secondly,the relatively large value of d indicates that the atomic size difference between components is too large for this alloy system to form entire solid-solution phases.Because the large atomic size mismatch could lead to serious lattice distortion and subsequently increase the corresponding strain energy which could lower the stability of solid-solution.Actually,the interstitial solubil-ity of carbon element in alloy is quite limited,so that carbon ele-ment has a strong tendency to exist in the form of carbonization or graphite in alloy.Moreover,the diffusion of atoms in the matrix could be suppressed due to large atomic size difference.Thus it facilitates the atomic segregation,even results in the formation of amorphous structures [35].As listed in Table 3,atomic radiuses of C (0.77Å)and Al (1.43Å)are significantly different from the other elements.This could be a reasonable explanation for the formation of the Cr 23C 6carbide and the Al-rich ordered BCC phase.In addition,it can also be found that the mixing enthalpy of most of the atomic pairs is highly negative (shown in Table 3).Al and C are the main contributor to the negative enthalpy of mixing in this alloy,indicat-ing their strong tendency for ordering or clustering.It is interesting to note that the mixing enthalpy of C and Cr (À61kJ/mol)is the most negative.This could be another factor which contributes to the formation of the Cr 23C 6carbide in the alloy.In addition,as above-mentioned,Fe has a high concentration (12.7±0.4at.%)in Cr 23C 6phase,revealing that Fe has high solubility in this type Cr 23C 6phase.According to Table 3,the mixing enthalpy of C and Fe (À50kJ/mol)stays a highly negative level,leading to Fe atoms preferring C sites.Mechanically alloyed powders of Al 0.5CrFeNiCo 0.3-C 0.2HEA exhibit simple solid solution structure within 38h of mill-ing,which can be attributed to the formation of supersaturated solid solution.The solid solubility extension can be ascribed to the high mixing entropy effect as well as the non-equilibrium state of the MA process [36].Interestingly,twinned FCC phase is also ob-served.Fig.6shows TEM images and corresponding SAED patterns of the FCC phase with nanoscale twins.It is worth pointing out that nanoscale twins are found only in the FCC phase,which has been confirmed by EDS/TEM and the corresponding diffraction patterns.The lamella thickness of the nanoscale twins shown in Fig.6(a)is less than 60nm.The corresponding SAED pattern (the matrix axisis [011]M and the twin axis is ½0 1 1 T)which is shown in the inset of the upper right of Fig.6(a)indicates the nanoscale twins belong to the FCC phase.Actually,existing researches in deformation twin-ning of nanocrystalline materials are mainly focus on FCC crystal structure metals,which is probably attributed to their favorable capability of deformation twinning [37].Fig.6(b)illustrates a noticeable twin structure of the FCC phase surrounding by grey phases.These grey phases are found to be the Cr 23C 6carbide through EDS/TEM analysis,revealing that the formation of Cr 23C 6might have effect on deformation twinning.Since Cr 23C 6shows a hard brittle texture [38],it could be presumed that partial FCC phase between the Cr 23C 6carbide is not readily deformed and con-solidated.Under the isostatic pressure of 30MPa during the SPS process,certain parallel crystal faces of partial crystals moving opposite to each other along a direction with a certain value of dis-placement distance.Thus twinning in the FCC phase may occur dur-ing the phase evolution and densification with the aim to be more stable and attaining complete densification [29].Moreover,a cer-tain twin system could be activated by a critical resolved shear stress which can be achieved during crystal deformation and phase evolution process.It is worth pointing out that similar nanoscale twins in CoNiFeAl 0.6Ti 0.4and CrCoNiFeAl 0.6Ti 0.4HEAs prepared by MA and SPS have been observed in previous studies of our research group [28,29].Table 3The chemical mixing enthalpy D H mix ij ;kJ =mol of binary equiatomic alloys calculated by Miedema’s approach [34].Element (atomic sizes,Å)C Ni Cr Co Fe Al C (0.77)–À39À61À42À50À36Ni (1.24)––À70À2À22Cr (1.25)–––À4À1À10Co (1.26)––––À1À19Fe (1.26)–––––À11Al (1.43)––––––S.Fang et al./Materials and Design 54(2014)973–9799773.3.Mechanical propertiesFig.7shows the room-temperature compressive stress–strain curve of bulk Al 0.5CrFeNiCo 0.3C 0.2HEA after SPS.The compressive strength and compression ratio of the alloy are 2131MPa and 3.0%,respectively.The average Vickers hardness of Al 0.5CrFeNiC-o 0.3C 0.2HEA after SPS has been measured to be 617±25HV.How-ever,plastic deformation behavior is not characterized in theindicating a limited compression plasticity of mechanical properties of Al 0.5CrFeNiCo 0.3C 0.2HEA HEAs of AlCrFeNiCo HEA system are listed The listed HEAs are prepared by casting 0.5CrFeNiCo 0.3C 0.2HEA studied in this paper.hardness of these as-cast HEAs has beenreported.Obviously,Al 0.5CrFeNiCo 0.3C 0.2alloy exhibits the highest hardness of the HEAs listed in Table 4.The high strength and high hardness of Al 0.5CrFeNiCo 0.3C 0.2HEA is possibly due to the formation of the ordered BCC phase and the Cr 23C 6carbide,the nanocrystalline structure,as well as solid solu-tion strengthening mechanism of Al atoms.It is worth pointing out that nanoscale twins might also have a considerable effect on strengthening the alloy.The strengthening mechanism of nano-scale twins is associated with the complicated interaction between dislocations and twin boundaries.It is believed that twin bound-aries are effective in blocking dislocation motion,especially when the thickness of twin/matrix lamellae decreases down to the nano-scale,and a Hall–Petch-type relationship exists for twin boundary strengthening [39,40].The limited compression ratio may owing to the brittle Cr 23C 6carbide,the ordered BCC phase,solid solution strengthening of Al atoms and ultrafine porosities.4.ConclusionsAl 0.5CrFeNiCo 0.3C 0.2high entropy alloy with nanocrystalline has been successfully synthesized by MA and SPS.A supersaturated so-lid solution with both FCC and BCC structures is evidently observed after MA.After SPS,Cr 23C 6carbide and an ordered BCC phase are newly formed.The TEM analysis results confirm that the alloy con-sists of one FCC phase,one BCC phase,Cr 23C 6carbide and one or-dered BCC pared with the nominal composition of Al 0.5CrFeNiCo 0.3C 0.2high entropy alloy,the FCC phase is enriched in Fe–Ni,the BCC phase is enriched in Ni–Al,while the ordered BCC phase is especially enriched in Al.The grain size of these phases shows a wide distribution ranging from several hundred nanometers to 1l m.Nanoscale deformation twins present only in partial FCC phase.The compressive strength,compression ratio and Vickers hardness of Al 0.5CrFeNiCo 0.3C 0.2high entropy alloy are 2131MPa,3.0%and 617±25HV,respectively.AcknowledgementsThe authors wish to acknowledge the financial support by National Natural Science Foundation of China (Grant No.51271080.)and Project supported by Guangdong Provincial Natural Science Foundation of China (Grant No.S2011010002227.).References[1]Zhao ZD,Chen Q,Chao HY,Huang SH.Microstructural evolution and tensilemechanical properties of thixoforged ZK60-Y magnesium alloys produced by two different routes.Mater Des 2010;31(4):1906–16.[2]Yeh JW,Chen SK,Lin SJ,Gan JY,Chin TS,Shun TT,et al.Nanostructured high-entropy alloys with multiple principal elements:novel alloy design concepts and outcomes.Adv Eng Mater 2004;6(5):299–303.and SAED pattern of twinned FCC phase.(a)a twinned FCC grain with corresponding SAED pattern along Z M =[01surrounded by Cr 23C 6carbide.7.Stress–strain curve of bulk Al 0.5CrFeNiCo 0.3C 0.2HEA under compression room temperature.Table 4Mechanical properties of HEAs at room temperature.AlloysProcess r y(MPa)r max(MPa)e p(%)Hardness (Hv)Refs.Al 0.5CrFeNiCo 0.3C 0.2SPS –2131 3.0617±25This work Al 0.5CrFeNiCo Arc-melting –––247[18]Al 0.9CrFeNiCo Arc-melting –––527[19]AlCrFeNiCoArc-melting–––495[19][3]Huang PK,Yeh JW,Shun TT,Chen SK.Multi-principal-element alloys withimproved oxidation and wear resistance for thermal spray coating.Adv Eng Mater2004;6(1–2):74–8.[4]Tong CJ,Chen YL,Chen SK,Yeh JW,Shun TT,Tsau CH,et al.Microstructurecharacterization of Al x CoCrCuFeNi high-entropy alloy system with multiprincipal elements.Metall Mater Trans A2005;36(4):881–93.[5]Wang YP,Li BS,Ren MX,Yang C,Fu HZ.Microstructure and compressiveproperties of AlCrFeCoNi high entropy alloy.Mater Sci Eng A2008;491(1–2): 154–8.[6]Li C,Li JC,Zhao M,Jiang Q.Effect of alloying elements on microstructure andproperties of multiprincipal elements high-entropy alloys.J Alloy Compd 2009;475(1–2):752–7.[7]Zhou YJ,Zhang Y,Wang YL,Chen GL.Microstructure and compressiveproperties of multicomponent Al x(TiVCrMnFeCoNiCu)100Àx high-entropy alloys.Mater Sci Eng A2007;454–455:260–5.[8]Varalakshmi S,Rao GA,Kamaraj M,Murty BS.Hot consolidation andmechanical properties of nanocrystalline equiatomic AlFeTiCrZnCu high entropy alloy after mechanical alloying.J Mater Sci2010;45(19):5158–63. [9]Varalakshmi S,Kamaraj M,Murty BS.Processing and properties ofnanocrystalline CuNiCoZnAlTi high entropy alloys by mechanical alloying.Mater Sci Eng A2010;527(4–5):1027–30.[10]Tong CJ,Chen MR,Chen SK,Yeh JW,Shun TT,Lin SJ,et al.Mechanicalperformance of the Al x CoCrCuFeNi high-entropy alloy system with multiprincipal elements.Metall Mater Trans A2005;36(5):1263–71.[11]Wu JM,Lin SJ,Yeh JW,Chen SK,Huang YS.Adhesive wear behavior ofAl x CoCrCuFeNi high-entropy alloys as a function of aluminum content.Wear 2006;261(5–6):513–9.[12]Tung CC,Yeh JW,Shun TT,Chen SK,Huang YS,Chen HC.On the elementaleffect of AlCoCrCuFeNi high-entropy alloy system.Mater Lett2007;61(1):1–5.[13]Zhang KB,Fu ZY,Zhang JY,Wang WM,Lee SW,Niihara K.Characterization ofnanocrystalline CoCrFeNiTiAl high-entropy solid solution processed by mechanical alloying.J Alloy Compd2010;495(1):33–8.[14]Praveen S,Murty BS,Kottada RS.Alloying behavior in multi-componentAlCoCrCuFe and NiCoCrCuFe high entropy alloys.Mater Sci Eng A2012;534: 83–9.[15]Wei SZ,Zhu JH,Xu LJ,Long R.Effects of carbon on microstructures andproperties of high vanadium high-speed steel.Mater Des2006;27(1):58–63.[16]Guo J,Shang CJ,Yang SW,Wang Y,Wang LW,He XL.Effect of carbon contenton mechanical properties and weather resistance of high performance bridge steels.J Iron Steel Res Int2009;16(6):63–9.[17]Chou HP,Chang YS,Chen SK,Yeh JW.Microstructure,thermophysical andelectrical properties in Al x CoCrFeNi(06x62)high-entropy alloys.Mater Sci Eng B2009;163(3):184–9.[18]Lin CM,Tsai HL.Evolution of microstructure,hardness,and corrosion propertiesof high-entropy Al0.5CoCrFeNi alloy.Intermetallics2011;19(3):288–94. [19]Wang WR,Wang WL,Wang SC,Tsai YC,Lai CH,Yeh JW.Effects of Al additionon the microstructure and mechanical property of Al x CoCrFeNi high-entropy alloys.Intermetallics2012;26:44–51.[20]Varalakshmi S,Kamaraj M,Murty BS.Synthesis and characterization ofnanocrystalline AlFeTiCrZnCu high entropy solid solution by mechanical alloying.J Alloy Compd2008;460(1–2):253–7.[21]Zhang KB,Fu ZY,Zhang JY,Shi J,Wang WM,Wang H,et al.NanocrystallineCoCrFeNiCuAl high-entropy solid solution synthesized by mechanical alloying.J Alloy Compd2009;485(1–2):L31–4.[22]Sriharitha R,Murty BS,Kottada RS.Phase formation in mechanically alloyedAl x CoCrCuFeNi(x=0.45,1, 2.5,5mol)high entropy alloys.Intermetallics 2013;32:119–26.[23]Chen WP,Fu ZQ,Fang SC,Xiao HQ,Zhu DZ.Alloying behavior,microstructureand mechanical properties in a FeNiCrCo0.3Al0.7high entropy alloy.Mater Des 2013;51:854–60.[24]Suryanarayana C.Mechanical alloying and milling.Prog Mater Sci2001;46(1–2):45–62.[25]Chen YL,Hu YH,Tsai CW,Hsieh CA,Kao SW,Yeh JW,et al.Alloying behavior ofbinary to octonary alloys based on Cu–Ni–Al–Co–Cr–Fe–Ti–Mo during mechanical alloying.J Alloy Compd2009;477(1–2):696–705.[26]Munir ZA,Anselmi-Tamburini U,Ohyanagi M.The effect of electricfield andpressure on the synthesis and consolidation of materials:a review of the spark plasma sintering method.J Mater Sci2006;41(3):763–77.[27]Fu ZQ,Chen WP,Xiao HQ,Zhou LW,Zhu DZ,Yang SF.Fabrication andproperties of nanocrystalline Co0.5FeNiCrTi0.5high entropy alloy by MA–SPS technique.Mater Des2013;44:535–9.[28]Fu ZQ,Chen WP,Fang SC,Zhang DY,Xiao HQ,Zhu DZ.Alloying behavior anddeformation twinning in a CoNiFeCrAl0.6Ti0.4high entropy alloy processed by spark plasma sintering.J Alloy Compd2013;553:316–23.[29]Chen WP,Fu ZQ,Fang SC,Wang YP,Xiao HQ,Zhu DZ.Processing,microstructure and properties of Al0.6CoNiFeTi0.4high entropy alloy with nanoscale twins.Mater Sci Eng A2013;565:439–44.[30]GB/T7314.Metallic Materials–Compression testing at ambient temperature.National Standard of the People’s Republic of China.2005.[31]Chen YL,Hu YH,Hsieh CA,Yeh JW,Chen petition between elementsduring mechanical alloying in an octonary multi-principal-element alloy system.J Alloy Compd2009;481(1–2):768–75.[32]Zhang Y,Zhou YJ,Lin JP,Chen GL,Liaw PK.Solid-solution phase formationrules for multi-component alloys.Adv Eng Mater2008;10(6):534–8.[33]Yang X,Zhang Y.Prediction of high-entropy stabilized solid-solution in multi-component alloys.Mater Chem Phys2012;132(2–3):233–8.[34]Takeuchi A,Inoue A.Classification of bulk metallic glasses by atomic sizedifference,heat of mixing and period of constituent elements and its application to characterization of the main alloying element.Mater Trans 2005;46(12):2817–29.[35]Zhang LC,Kim KB,Yu P,Zhang WY,Kunz U,Eckert J.Amorphization inmechanically alloyed(Ti,Zr,Nb)–(Cu,Ni)–Al equiatomic alloys.J Alloy Compd 2007;428(1):157–63.[36]Sui HX,Zhu M,Qi M,Li GB,Yang DZ.The enhancement of solid solubility limitsof AlCo intermetallic compound by high-energy ball milling.J Appl Phys1992;71(6):2945–9.[37]Zhu YT,Liao XZ,Wu XL.Deformation twinning in nanocrystalline materials.Prog Mater Sci2012;57(1):1–62.[38]Hirota K,Mitani K,Yoshinaka M,Yamaguchi O.Simultaneous synthesisand consolidation of chromium carbides(Cr3C2,Cr7C3and Cr23C6)by pulsed electric-current pressure sintering.Mater Sci Eng A2005;399(1–2): 154–60.[39]Youngdahl CJ,Weertman JR,Hugo RC,Kung HH.Deformation behavior innanocrystalline copper.Scr Mater2001;44(8–9):1475–8.[40]Yan FK,Liu GZ,Tao NR,Lu K.Strength and ductility of316L austenitic stainlesssteel strengthened by nanoscale twin bundles.Acta Mater2012;60(3): 1059–71.S.Fang et al./Materials and Design54(2014)973–979979。
Ti_ZrN_瓷界面微观结构和力学性能_段珍珍
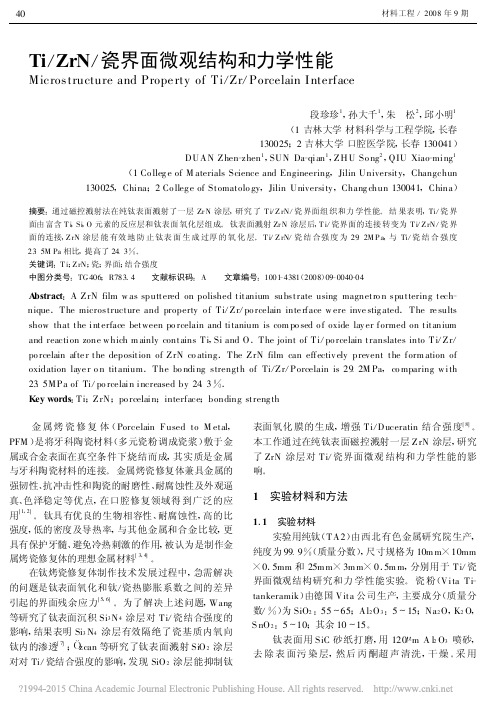
Ti/ZrN/瓷界面微观结构和力学性能Microstructure and Prope rty of Ti/Zr/Porcelain Interface段珍珍1,孙大千1,朱 松2,邱小明1(1吉林大学材料科学与工程学院,长春130025;2吉林大学口腔医学院,长春130041)DUAN Zhen-zhen1,SUN Da-qian1,ZH U So ng2,QIU Xiao-ming1 (1Co lleg e of M aterials Science and Engineering,Jilin University,Changchun 130025,China;2Co lleg e of Stomatolo gy,Jilin University,Chang chun130041,China)摘要:通过磁控溅射法在纯钛表面溅射了一层Zr N涂层,研究了T i/Z rN/瓷界面组织和力学性能。
结果表明,Ti/瓷界面由富含T i,Si,O元素的反应层和钛表面氧化层组成。
钛表面溅射Zr N涂层后,T i/瓷界面的连接转变为T i/Z rN/瓷界面的连接,Z rN涂层能有效地防止钛表面生成过厚的氧化层。
T i/Z rN/瓷结合强度为29.2M P a,与Ti/瓷结合强度23.5M Pa相比,提高了24.3%。
关键词:T i;Z rN;瓷;界面;结合强度中图分类号:TG406;R783.4 文献标识码:A 文章编号:1001-4381(2008)09-0040-04A bstract:A ZrN film w as sputtered on polished titanium substrate using magnetro n sputtering tech-nique.The microstructure and property o f Ti/Zr/po rcelain inte rface w ere inve stig ated.The re sults show that the interface between po rcelain and titanium is com po sed o f oxide lay er formed on titanium and reaction zone w hich m ainly contains Ti,Si and O.The joint of Ti/po rcelain translates into Ti/Zr/ po rcelain afte r the deposition of ZrN co ating.The ZrN film can effectiv ely prevent the form ation of oxidation laye r o n titanium.The bo nding strength of Ti/Zr/Porcelain is29.2M Pa,co mparing w ith 23.5MPa of Ti/po rcelain increased by24.3%.Key words:Ti;ZrN;po rcelain;interface;bonding streng th 金属烤瓷修复体(Porcelain Fused to M etal, PFM)是将牙科陶瓷材料(多元瓷粉调成瓷浆)敷于金属或合金表面在真空条件下烧结而成,其实质是金属与牙科陶瓷材料的连接。
碳酸化预处理对钢渣-水泥复合胶凝材料体积安定性及水化活性的影响

第42卷第3期2023年3月硅㊀酸㊀盐㊀通㊀报BULLETIN OF THE CHINESE CERAMIC SOCIETYVol.42㊀No.3March,2023碳酸化预处理对钢渣-水泥复合胶凝材料体积安定性及水化活性的影响房延凤1,王凇宁1,佟㊀钰1,孙小巍1,丁向群1,苏㊀文2(1.沈阳建筑大学材料科学与工程学院,沈阳㊀110168;2.中国建筑土木建设有限公司,北京㊀100070)摘要:钢渣水化活性差,体积安定性不良限制了其作为辅助性胶凝材料的应用,但钢渣具有很好的碳酸化活性㊂本文在对钢渣进行预处理的过程中通过调整CO2浓度及碳酸化时间,调控钢渣的碳酸化程度,分析了碳酸化对钢渣微观结构及固碳效果的影响,同时评价了碳酸化钢渣作为辅助性胶凝材料的可行性㊂结果表明:含30%(质量分数)钢渣的水泥砂浆试块3㊁28d抗压强度较未掺钢渣水泥砂浆分别降低了43.2%和30.0%,净浆试块经压蒸试验后由于膨胀过大而溃散;CO2浓度对钢渣的固碳量有显著的影响,高浓度(体积分数为99.9%)CO2进行碳化养护3min时钢渣固碳量就达到了3.67%㊂钢渣的体积安定性与碳酸化程度呈正相关,而过度碳酸化处理会降低其水化活性,掺加30%(质量分数)碳酸化预处理3㊁10min钢渣的砂浆3d抗压强度较掺加30%原始钢渣的砂浆分别提高了28.3%和15.8%㊂关键词:钢渣;碳酸化;复合胶凝材料;水化活性;体积安定性;抗压强度中图分类号:TQ172㊀㊀文献标志码:A㊀㊀文章编号:1001-1625(2023)03-1001-07 Effect of Carbonation Pretreatment on Volume Stability andHydration Activity of Steel Slag-Cement CompositeCementitious MaterialsFANG Yanfeng1,WANG Songning1,TONG Yu1,SUN Xiaowei1,DING Xiangqun1,SU Wen2(1.School of Materials Science and Engineering,Shenyang Jianzhu University,Shenyang110168,China;2.China Construction Civil Engineering Co.,Ltd.,Beijing100070,China)Abstract:The application of steel slag as supplementary cementitious materials is limited by its poor hydration activity and volume stability,while steel slag shows excellent carbonation activity.In this paper,the carbonation degree of steel slag was controlled by adjusting the CO2concentration and carbonation time.The effect of carbonation on the microstructure and CO2uptake of steel slag was analyzed,and the volume stability and hydration activity of carbonated steel slag used as supplementary cementitious materials were evaluated.The results show that the3,28d compressive strength of mortar containing30%(mass fraction)steel slag reduce by43.2%and30.0%,respectively,compared to the mortar without steel slag.And paste specimen collapses due to expansion after autoclaving experiment.The CO2uptake of steel slag is significantly affected by the concentration of CO2.The CO2uptake of steel slag arrives at3.67%after carbonation for3min at high concentration(volume fraction is99.9%)of CO2.The volume stability of steel slag is positively correlated with the degree of carbonation,while over carbonation treatment reduces the hydration activity.The3d compressive strength of mortar containing30%(mass fraction)steel slag carbonated for3,10min increases by28.3%and15.8%,respectively. Key words:steel slag;carbonation;composite cementitious material;hydration activity;volume stability;compressive strength收稿日期:2022-10-16;修订日期:2022-11-30基金项目:国家自然科学基金青年科学基金项目(51808354);中国博士后科学基金资助项目(2018M641712);辽宁省教育厅科学研究经费项目(lnjc202017)作者简介:房延凤(1988 ),女,博士,副教授㊂主要从事工业废渣建材资源化利用及特种水泥方面的研究㊂E-mail:fangyf@1002㊀资源综合利用硅酸盐通报㊀㊀㊀㊀㊀㊀第42卷0㊀引㊀言中国是世界上最大的钢铁生产国家,产量超过全球总产量的50%[1]㊂钢铁行业不仅排放大量的CO2,还会产生大量无法有效利用的钢渣(约占粗钢产量的15%~20%)[2]㊂目前中国钢渣累积存量超过18亿吨[3],长期堆存不仅造成资源的浪费,且易产生粉尘,污染附近水域,从而引起一系列环境问题[4-5]㊂建材资源化利用是消纳钢渣的有效途径,但钢渣存在成分复杂多变㊁体积安定性不良㊁水化活性差等问题,用作辅助胶凝材料或者骨料存在一系列安全问题,限制了其在建筑材料中的应用[6-9]㊂钢渣虽然水化活性和体积安定性差,但具有较好的碳酸化活性[10-11],尤其是钢渣中的f-CaO和f-MgO可以快速与CO2反应[12],钢渣经过碳酸化养护,可以制备安定性良好的钢渣制品[13-16],具有很好的应用前景㊂Wang等[17]采用半干法对钢渣进行碳酸化处理,研究发现通过调控碳酸化工艺可以调控碳酸化产物的种类和粒度㊂同时很多研究[18-20]表明,纳米碳酸钙和纳米水化硅酸钙(C-S-H)在水泥水化的过程中能够促进早期水化㊂因此,若能通过调控钢渣的碳酸化程度及反应产物的种类㊁粒度从而达到改善钢渣体积安定性和水化活性的目的,则可促进钢渣作为辅助性胶凝材料的广泛应用,从而大规模消解堆存的钢渣,同时固定与储存温室气体CO2㊂本研究通过调整CO2浓度及碳酸化时间,研究了碳酸化对钢渣-水泥复合胶凝材料体积安定性和水化活性的影响,同时评价了碳酸化钢渣作为辅助性胶凝材料的可行性㊂1㊀实㊀验1.1㊀原材料试验所用钢渣为本钢集团的转炉钢渣经粉碎㊁粉磨㊁筛分所得,比表面积和密度分别为320m2/kg和3.25g/cm3;普通硅酸盐水泥(P㊃O42.5)的比表面积和密度分别为350m2/kg和3.06g/cm3㊂砂为标准砂㊂钢渣和水泥的主要化学成分如表1所示㊂由表1可知,钢渣中的CaO含量较水泥中低,Fe2O3和MgO含量偏高㊂表1㊀钢渣和水泥的主要化学组成Table1㊀Main chemical composition of steel slag and cementRaw material Mass fraction/%CaO SiO2Fe2O3P2O3MgO Al2O3MnO TiO2CrO2SO3 Steel slag36.414.825.2 2.012.6 5.0 1.00.70.60.3 Cement61.121.5 2.9 2.1 5.2 2.51.2㊀试验设计本研究旨在探索钢渣作为辅助性胶凝材料替代部分水泥的可能性,设计了不同钢渣掺量㊂同时在不同条件下对钢渣进行碳酸化预处理,用碳酸化的钢渣替代30%(质量分数)水泥分析其对力学性能和体积安定性的影响㊂将钢渣与8%(质量分数)的水混合并搅拌均匀,然后将钢渣放入反应釜进行碳酸化预处理㊂碳酸化过程中的CO2压力为0.2MPa,体积分数分别为99.9%和20.0%,碳酸化时间控制在3㊁10min㊂砂浆和净浆试块的配合比及试块尺寸等信息如表2所示㊂在砂浆制备过程中,砂胶比和水胶比分别为3.0和0.5,净浆制备过程中水胶比为0.3㊂砂浆和净浆试块在成型24h后脱模,然后养护至相应龄期进行测试㊂表2㊀试验设计Table2㊀Experiments designSpecimen Steel slagcontent/%Mortar PasteSize/(mmˑmmˑmm)Number Size/(mmˑmmˑmm)NumberSS00 SS1010 SS2020 SS3030 CSS-130 CSS-230 CSS-33040ˑ40ˑ16040ˑ40ˑ16040ˑ40ˑ16040ˑ40ˑ16040ˑ40ˑ16040ˑ40ˑ16040ˑ40ˑ160999999925ˑ25ˑ28025ˑ25ˑ28025ˑ25ˑ28025ˑ25ˑ28025ˑ25ˑ28025ˑ25ˑ28025ˑ25ˑ2803333333CSS-43040ˑ40ˑ160925ˑ25ˑ2803㊀㊀注:CSS-1㊁CSS-2㊁CSS-3和CSS-4所掺加的钢渣分别在99.9%的CO2中处理3㊁10min和20.0%的CO2中处理3㊁10min㊂第3期房延凤等:碳酸化预处理对钢渣-水泥复合胶凝材料体积安定性及水化活性的影响1003㊀1.3㊀测试方法和仪器按照‘水泥胶砂强度检验方法(ISO 法)“(GB /T 17671 2021)测定水泥砂浆试块的抗折强度和抗压强度,参考‘水泥压蒸安定性试验方法“(GB /T 750 1992)测试体积安定性㊂使用布鲁克D8Advance X 射线衍射仪在40kV 和40mA 下进行物相分析,步长为0.04ʎ㊂使用梅特勒-托利多TG /DSC1系统测试碳酸化钢渣在不同碳酸化条件下的固碳量㊂采用扫描电镜(FE-SEM,FEINOVA Nano SEM 450)分析碳酸化预处理对钢渣微观结构的影响㊂2㊀结果与讨论2.1㊀钢渣掺量对砂浆抗压强度和体积安定性的影响不同钢渣掺量砂浆的3㊁7㊁28d 抗折强度和抗压强度结果如图1所示㊂从图1中可以看出,随着钢渣掺量的增加,试块的抗折强度和抗压强度显著降低,其中对3d 力学性能影响更加明显㊂SS10㊁SS20和SS30的3d 抗压强度与SS0相比分别降低了22.3%㊁30.0%和43.2%,而SS10㊁SS20和SS30的28d 抗压强度较SS0分别降低了11.1%㊁21.7%和30.0%㊂可以看出,掺加钢渣对钢渣-水泥复合胶凝材料体系早期强度的发展具有显著的不利影响㊂图1㊀钢渣掺量对砂浆抗折强度和抗压强度的影响Fig.1㊀Effect of steel slag content on flexural strength and compressive strength of mortar 钢渣中含有f-CaO 和f-MgO,这会对硬化浆体的体积安定性产生不利影响㊂通过测试净浆试块的初始尺寸和经过高压釜压蒸3h 后的尺寸,计算线膨胀率,结果如表3所示㊂结果表明,膨胀率随着钢渣含量的增加而逐渐增大㊂SS10的膨胀率为0.47%,略低于标准限值(0.5%)㊂当钢渣含量达到30%时,膨胀过大导致试块溃散,无法得到膨胀率,SS30压蒸后的外观形貌如图2(a)所示㊂图2(b)为SS30压蒸试验前后的TG-DTG 曲线,可以看出经过3h 的压蒸后Ca(OH)2和Mg(OH)2的含量分别达到12.30%和4.17%(质量分数),而压蒸前并未观察到Mg (OH)2的失重,Ca (OH)2的含量也仅为5.22%,说明压蒸过程加速了f-CaO 和f-MgO 的水化反应㊂表3㊀不同钢渣掺量净浆试块的线膨胀率Table 3㊀Linear expansion rate of paste containing different steel slag contentSpecimen SS0SS10SS20SS30Linear expansion rate /%0.060.47 1.212.2㊀碳酸化预处理对钢渣固碳量及微观形貌的影响图3为不同条件下碳酸化预处理钢渣的XRD 谱,SS 表示未碳酸化处理钢渣,CSS1和CSS2分别表示用99.9%浓度的CO 2碳酸化3㊁10min 的钢渣,CSS3和CSS4分别表示用20.0%浓度的CO 2碳酸化3㊁10min 的钢渣㊂从图3中可以看出,经过碳酸化预处理后硅酸二钙(C 2S)的衍射峰强度明显降低,而方解石的衍射峰强度显著增强㊂文献[21-22]表明钢渣在加速碳酸化过程中也会形成结晶度差的CaCO 3㊁文石㊁球霰石和1004㊀资源综合利用硅酸盐通报㊀㊀㊀㊀㊀㊀第42卷方解石,但在XRD 分析中只观察到方解石的衍射峰㊂CaCO 3种类的差异可能是钢渣的矿物组成不同造成的㊂γ-C 2S 碳化过程中很容易形成球霰石,而β-C 2S 易碳酸化形成方解石[8,17]㊂碳酸化钢渣中铁铝酸四钙(C 4AF)的衍射峰强度没有明显降低,说明C 4AF 的碳酸化反应活性较低㊂图2㊀SS30压蒸后的外观形貌和TG-DTG 曲线Fig.2㊀Appearance and TG-DTG curves of SS30afterautoclaving 图3㊀不同碳酸化条件下钢渣的XRD 谱Fig.3㊀XRD patterns of steel slag carbonated at differentconditions 图4㊀不同碳酸化条件下钢渣的TG-DTG 曲线及固碳量Fig.4㊀TG-DTG curves and CO 2uptake of steel slag carbonated at different conditions 对钢渣和碳酸化预处理的钢渣进行TG 分析,以评价其固碳结果,结果如图4所示㊂可以看出质量损失主要集中在400~450ħ和500~850ħ,分别是Ca(OH)2和CaCO 3的分解造成的㊂钢渣碳酸化过程中形成的CaCO 3与天然CaCO 3相比,分解温度较低,为500~850ħ[17]㊂根据TG 曲线计算得到的固碳量也列于图4中㊂碳酸化过程中的CO 2浓度和碳酸化时间对碳酸化效果影响较大,用20.0%浓度的CO 2碳酸化3㊁10min 的钢渣中CO 2含量仅为1.2%和2.5%(质量分数,下同),相对应地,固碳量分别为0.27%和1.57%;而用高浓度CO 2进行碳酸化养护3㊁10min时,CO 2含量达到4.6%和5.9%,此时固碳量分别为3.67%和4.97%㊂在不同CO 2浓度下,碳酸化预处理不同时间的钢渣的SEM 照片如图5(a)~(d)所示㊂尽管在图3和图4的XRD 谱和TG 曲线中表明,碳酸化3min 就已生成大量方解石,但在SEM 照片中未发现具有特定形第3期房延凤等:碳酸化预处理对钢渣-水泥复合胶凝材料体积安定性及水化活性的影响1005㊀态的结晶良好的碳酸钙,其原因可能是在碳酸化过程早期的碳酸钙颗粒粒径较小或为结晶度差的无定形碳酸钙㊂随着碳酸化时间的增加,碳酸钙的粒径逐渐增大㊂CO 2压力对碳酸钙的颗粒尺寸有明显影响,低浓度CO 2有利于碳酸钙颗粒尺寸的增大㊂图5㊀不同碳酸化条件下钢渣的SEM 照片Fig.5㊀SEM images of steel slag carbonated at different conditions 2.3㊀碳酸化预处理对钢渣水化活性的影响将含有30%钢渣和碳酸化钢渣的砂浆试块养护至3㊁7㊁28d 后测试其抗折强度和抗压强度,结果如图6所示㊂碳酸化预处理对钢渣的水化活性有显著影响,适当的碳酸化预处理可有效提高钢渣的水化活性㊂与对照组SS30相比,CSS-1和CSS-2的3d 抗压强度分别提高了28.3%和15.8%,而28d 抗压强度分别提高了13.4%和10.8%㊂因此碳酸化钢渣可以加速水化进程,改善砂浆的早期力学性能㊂在低浓度(20.0%)CO 2下对钢渣进行碳酸化预处理,碳酸化效果较差,对钢渣胶凝活性的提升作用并不明显,CSS-3和CSS-4的3d 抗压强度分别为对照组SS30的108.9%和96.0%,这是由于低浓度CO 2条件下碳酸化程度较低,生成的C-S-H 和CaCO 3数量较少,且CaCO 3颗粒尺寸较大,晶核效应较弱㊂图6㊀碳酸化预处理钢渣对砂浆抗折强度和抗压强度的影响Fig.6㊀Effect of carbonation pretreated steel slag on flexural strength and compressive strength of martor1006㊀资源综合利用硅酸盐通报㊀㊀㊀㊀㊀㊀第42卷2.4㊀碳酸化预处理对钢渣体积安定性的影响图7和表4分别为掺加碳酸化钢渣净浆试块经3h 的压蒸试验后的外观形貌和膨胀率,可以看出经碳酸化预处理后,体积安定性得到了有效改善㊂即使用20.0%浓度的CO 2处理3min,体积安定性也较原钢渣有明显的提升,处理10min 后试块经压蒸后表面未出现任何裂纹,但相应砂浆试块的抗压强度为参比样的94.5%,尽管在此条件下钢渣水化活性稍有降低,但解决了钢渣体积安定性不良的问题㊂图7㊀含碳酸化钢渣的净浆试块压蒸后外观形貌Fig.7㊀Appearance of paste containing carbonated steel slag after autoclaving表4㊀碳酸化钢渣对净浆试块膨胀率的影响Table 4㊀Effect of carbonated steel slag on expansion rate of pasteSpecimen CSS-1CSS-2CSS-3CSS-4Expansion rate /%0.520.25 0.433㊀结㊀论1)钢渣的掺加对砂浆试块早期强度发展的不利影响更为明显㊂钢渣中的f-CaO 和f-MgO 会对硬化浆体的体积安定性产生不利影响,掺加30%钢渣的试样在经过3h 的压蒸试验后由于膨胀过大而溃散㊂2)碳酸化过程中的CO 2浓度和碳酸化时间对钢渣的固碳量影响较大,用低浓度(体积分数20.0%)CO 2对钢渣进行碳酸化预处理3㊁10min 后的固碳量仅为0.27%和1.57%,而用高浓度(体积分数99.9%)CO 2进行碳酸化养护3min 时其固碳量就达到了3.67%㊂碳酸化过程中形成了方解石,且20.0%CO 2浓度下生产的方解石尺寸较大㊂3)钢渣的碳酸化预处理对早期水化和钢渣的体积安定性有显著影响㊂适量的碳酸化可提高钢渣的水化活性,过度碳酸化反而会降低水化活性㊂掺加30%用高浓度(99.9%)CO 2碳酸化预处理3㊁10min 钢渣的砂浆3d 抗压强度较掺30%原始钢渣的矿浆分别提高了28.3%和15.8%,这归因于碳酸化过程中形成的C-S-H 和方解石,为水泥水化提供了形核位点㊂在低浓度(20.0%)CO 2下对钢渣的碳酸化效果不佳,对钢渣胶凝活性的提升作用并不明显㊂钢渣的体积安定性与碳酸化程度呈正相关,因此需要通过调整碳酸化条件来获得体积安定性和水化活性的平衡㊂参考文献[1]㊀SHU K Q,SASAKI K.Occurrence of steel converter slag and its high value-added conversion for environmental restoration in China:a review[J].Journal of Cleaner Production,2022,373:133876㊀第3期房延凤等:碳酸化预处理对钢渣-水泥复合胶凝材料体积安定性及水化活性的影响1007 [2]㊀张长森,李㊀杨,胡志超,等.钠盐激发钢渣水泥的早期水化特性及动力学[J].建筑材料学报,2021,24(4):710-715.ZHANG C S,LI Y,HU Z C,et al.Early hydration properties and kinetics of steel slag-cement activated by sodium salts[J].Journal of Building Materials,2021,24(4):710-715(in Chinese).[3]㊀WANG X B,LI X Y,YAN X,et al.Environmental risks for application of iron and steel slags in soils in China:a review[J].Pedosphere,2021,31(1):28-42.[4]㊀GUO J L,BAO Y P,WANG M.Steel slag in China:treatment,recycling,and management[J].Waste Management,2018,78:318-330.[5]㊀LAI M H,ZOU J J,YAO B Y,et al.Improving mechanical behavior and microstructure of concrete by using BOF steel slag aggregate[J].Construction and Building Materials,2021,277:122269.[6]㊀LE D H,SHEEN Y N,BUI Q B.An assessment on volume stabilization of mortar with stainless steel slag sand[J].Construction and BuildingMaterials,2017,155:200-208.[7]㊀WANG G,WANG Y H,GAO Z e of steel slag as a granular material:volume expansion prediction and usability criteria[J].Journal ofHazardous Materials,2010,184(1/2/3):555-560.[8]㊀FANG Y F,SU W,ZHANG Y Z,et al.Effect of accelerated precarbonation on hydration activity and volume stability of steel slag as asupplementary cementitious material[J].Journal of Thermal Analysis and Calorimetry,2022,147(11):6181-6191.[9]㊀JIANG Y,LING T C,SHI C J,et al.Characteristics of steel slags and their use in cement and concrete:a review[J].Resources,Conservationand Recycling,2018,136:187-197.[10]㊀BACIOCCHI R,COSTA G,DI GIANFILIPPO M,et al.Thin-film versus slurry-phase carbonation of steel slag:CO2uptake and effects onmineralogy[J].Journal of Hazardous Materials,2015,283:302-313.[11]㊀UKWATTAGE N L,RANJITH P G,LI X.Steel-making slag for mineral sequestration of carbon dioxide by accelerated carbonation[J].Measurement,2017,97:15-22.[12]㊀常㊀钧,吴昊泽.钢渣碳化机理研究[J].硅酸盐学报,2010,38(7):1185-1190.CHANG J,WU H Z.Study on carbonation mechanism of steel slag[J].Journal of the Chinese Ceramic Society,2010,38(7):1185-1190(in Chinese).[13]㊀LI L F,ZHONG X Z,LING T C.Effects of accelerated carbonation and high temperatures exposure on the properties of EAFS and BOFS pressedblocks[J].Journal of Building Engineering,2022,45:103504.[14]㊀LUO Z T,WANG Y,YANG G J,et al.Effect of curing temperature on carbonation behavior of steel slag compacts[J].Construction andBuilding Materials,2021,291:123369.[15]㊀YANG S,MO L W,DENG M.Effects of ethylenediamine tetra-acetic acid(EDTA)on the accelerated carbonation and properties of artificialsteel slag aggregates[J].Cement and Concrete Composites,2021,118:103948.[16]㊀MO L W,ZHANG F,DENG M,et al.Accelerated carbonation and performance of concrete made with steel slag as binding materials andaggregates[J].Cement and Concrete Composites,2017,83:138-145.[17]㊀WANG D,FANG Y F,ZHANG Y Y,et al.Changes in mineral composition,growth of calcite crystal,and promotion of physico-chemicalproperties induced by carbonation ofβ-C2S[J].Journal of CO2Utilization,2019,34:149-162.[18]㊀唐芮枫,崔素萍,王子明,等.钙硅摩尔比对纳米水化硅酸钙晶种早强作用的影响及机理[J].硅酸盐学报,2022,50(6):1626-1633.TANG R F,CUI S P,WANG Z M,et al.Effect of calcium-silicon molar ratio on early strength enhancement of nano-sized calcium silicate hydrate seeds[J].Journal of the Chinese Ceramic Society,2022,50(6):1626-1633(in Chinese).[19]㊀PEDROSA H C,REALES O M,REIS V D,et al.Hydration of Portland cement accelerated by C-S-H seeds at different temperatures[J].Cement and Concrete Research,2020,129:105978.[20]㊀BENTZ D P,ARDANI A,BARRETT T,et al.Multi-scale investigation of the performance of limestone in concrete[J].Construction andBuilding Materials,2015,75:1-10.[21]㊀CHANG J,WANG D,FANG Y F.Effects of mineralogical changes in BOFS during carbonation on pH and Ca and Si leaching[J].Constructionand Building Materials,2018,192:584-592.[22]㊀ZHANG H N,DONG J P,WEI C.Material properties of cement doped with carbonated steel slag through the slurry carbonation process:effectand quantitative model[J].Metallurgical and Materials Transactions B,2022,53(3):1681-1690.。
钢渣作为混合材在复合水泥中的应用
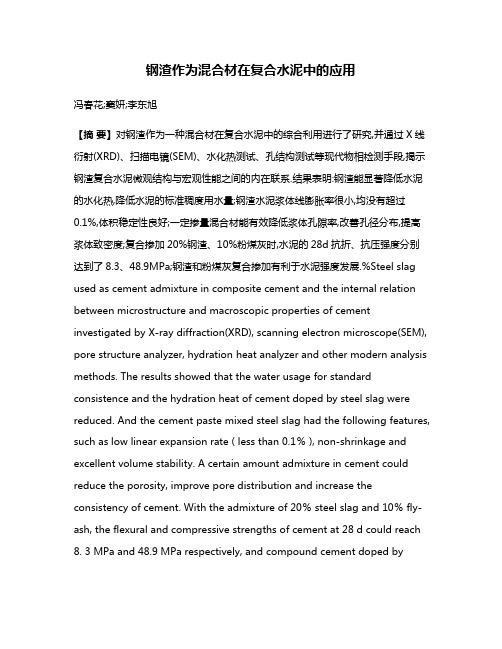
钢渣作为混合材在复合水泥中的应用冯春花;窦妍;李东旭【摘要】对钢渣作为一种混合材在复合水泥中的综合利用进行了研究,并通过X线衍射(XRD)、扫描电镜(SEM)、水化热测试、孔结构测试等现代物相检测手段,揭示钢渣复合水泥微观结构与宏观性能之间的内在联系.结果表明:钢渣能显著降低水泥的水化热,降低水泥的标准稠度用水量;钢渣水泥浆体线膨胀率很小,均没有超过0.1%,体积稳定性良好;一定掺量混合材能有效降低浆体孔隙率,改善孔径分布,提高浆体致密度;复合掺加20%钢渣、10%粉煤灰时,水泥的28d抗折、抗压强度分别达到了8.3、48.9MPa;钢渣和粉煤灰复合掺加有利于水泥强度发展.%Steel slag used as cement admixture in composite cement and the internal relation between microstructure and macroscopic properties of cement investigated by X-ray diffraction(XRD), scanning electron microscope(SEM), pore structure analyzer, hydration heat analyzer and other modern analysis methods. The results showed that the water usage for standard consistence and the hydration heat of cement doped by steel slag were reduced. And the cement paste mixed steel slag had the following features, such as low linear expansion rate ( less than 0.1% ), non-shrinkage and excellent volume stability. A certain amount admixture in cement could reduce the porosity, improve pore distribution and increase the consistency of cement. With the admixture of 20% steel slag and 10% fly-ash, the flexural and compressive strengths of cement at 28 d could reach 8. 3 MPa and 48.9 MPa respectively, and compound cement doped bysteel slag and fly ash was contributed to the strength development of cement efficiently.【期刊名称】《南京工业大学学报(自然科学版)》【年(卷),期】2011(033)001【总页数】6页(P74-79)【关键词】钢渣;复合水泥;混合材;孔结构;水化热【作者】冯春花;窦妍;李东旭【作者单位】南京工业大学,材料科学与工程学院,材料化学工程国家重点实验室,江苏,南京,210009;南京工业大学,材料科学与工程学院,材料化学工程国家重点实验室,江苏,南京,210009;南京工业大学,材料科学与工程学院,材料化学工程国家重点实验室,江苏,南京,210009【正文语种】中文【中图分类】TQ177.3钢渣是炼钢过程中产生的副产品。
材料科学与工程专业英语第二版_翻译答案(匡少平),单元:1,2,4,5,7,8,9,10,11,13,16,19,22
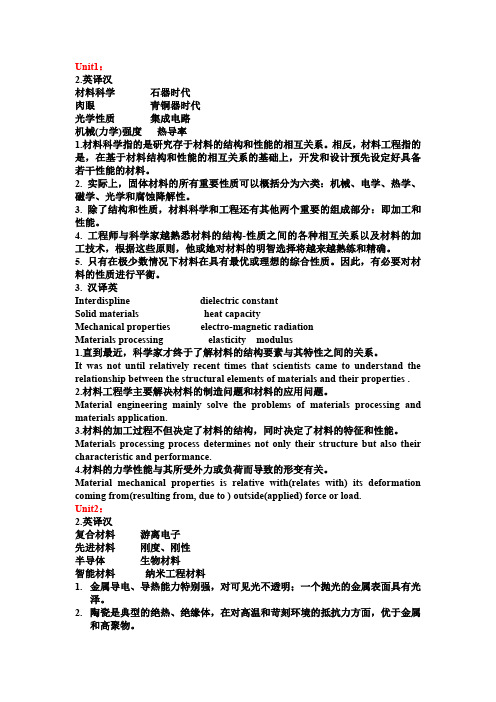
Unit1:2.英译汉材料科学石器时代肉眼青铜器时代光学性质集成电路机械(力学)强度热导率1.材料科学指的是研究存于材料的结构和性能的相互关系。
相反,材料工程指的是,在基于材料结构和性能的相互关系的基础上,开发和设计预先设定好具备若干性能的材料。
2. 实际上,固体材料的所有重要性质可以概括分为六类:机械、电学、热学、磁学、光学和腐蚀降解性。
3. 除了结构和性质,材料科学和工程还有其他两个重要的组成部分:即加工和性能。
4. 工程师与科学家越熟悉材料的结构-性质之间的各种相互关系以及材料的加工技术,根据这些原则,他或她对材料的明智选择将越来越熟练和精确。
5. 只有在极少数情况下材料在具有最优或理想的综合性质。
因此,有必要对材料的性质进行平衡。
3. 汉译英Interdispline dielectric constantSolid materials heat capacityMechanical properties electro-magnetic radiationMaterials processing elasticity modulus1.直到最近,科学家才终于了解材料的结构要素与其特性之间的关系。
It was not until relatively recent times that scientists came to understand the relationship between the structural elements of materials and their properties . 2.材料工程学主要解决材料的制造问题和材料的应用问题。
Material engineering mainly solve the problems of materials processing and materials application.3.材料的加工过程不但决定了材料的结构,同时决定了材料的特征和性能。
- 1、下载文档前请自行甄别文档内容的完整性,平台不提供额外的编辑、内容补充、找答案等附加服务。
- 2、"仅部分预览"的文档,不可在线预览部分如存在完整性等问题,可反馈申请退款(可完整预览的文档不适用该条件!)。
- 3、如文档侵犯您的权益,请联系客服反馈,我们会尽快为您处理(人工客服工作时间:9:00-18:30)。
P ro cedia Engineering 36 ( 2012 )292 – 2981877-7058 © 2012 Published by Elsevier Ltd.do i: 10.1016/j.proeng.2012.03.043Available online at Open access under CC BY -NC-ND license .293X. Yang et al. / P rocedia Engineering 36 ( 2012 )292 – 298with excellent properties such as high strength, better room temperature ductility, good resistances to wearand high thermal stability, but few HEAs can meet the requirements of aerospace industry [4-10].According to empirical rule, a rapid decrease in strength of HEAs occurs at temperatures above0.6T m, where T m is the melting temperature. To date, HEAs research has emphasized systems mainly based on the transition metals such as Cr, Mn, Fe, Co, Ni, Ti and Cu, and hardly any HEAs can be used atthe temperature above 1273K [4, 10]. In view of the excellent softening resistance, it is reasonable to explore high entropy alloys with high melting point. Recently American researchers have explored some refractory HEAs, composed of some transition metal elements with high melting temperature such as Ta,W, Nb, Mo and V [11, 12]. However, these alloys exhibit high density and low plastic strain. In this work,the lighter elements, Al and Ti, are selected to decrease the density and improve the ductility, thus the novel refractory HEAs, NbTiVTaAlx, are explored and their microstructure and mechanical properties are investigated.2.Experimental procedureAlloy ingots with nominal composition of NbTiVTaAl x (x values in molar ratio, x = 0, 0.2, 0.5, and1.0, denoted by Al0, Al0.2, Al0.5 and Al1.0, respectively) were prepared by arc melting the mixtures ofhigh-purity metals with the purity better than 99 wt% under a Ti-gettered high-purity argon atmosphereon a water-cooled Cu hearth. The alloys were remelted several times and flipped each times in order to improve homogeneity. The prepared alloy buttons with about 11 mm thick and 30 mm in diameter werecut into appropriate form for investigating their microstructure and compressive properties.Microstructure investigations of alloys were carried out by X-ray diffraction (XRD) using a PHILIPSAPD-10 diffractometer with Cu KĮ radiation. Cylindrical samples of ĭ3 mm × 6 mm were prepared forroom compressive tests and investigated using MTS 809 materials testing machine at room temperaturewith a strain rate of 2 × 10í4 sí1. The morphologies of cross sections and fracture surfaces were examined using a ZEISS SUPRA 55 scanning electron microscope (SEM) with energy dispersive spectrometry (EDS).3.Results and discussionFig. 1 (a) XRD patterns of the as-solidified NbTiVTaAl x (x=0, 0.25, 0.5, and 1) alloys; (b) the detailed scans for the peaks of (110)of BCC solid solutions.294X. Yang et al. / P rocedia Engineering 36 ( 2012 )292 – 298 Figure 1(a) and (b) show the XRD patterns of the specimens for NbTiVTaAl x alloy series. From Fig. 1(a), only BCC solid solution phase can be detected in all alloys, indicating that the addition of Al has little effect on the phase constitution of this type of HEAs, and the crystal-plane indices of BCC structure corresponding to diffraction peaks can be identified and marked in XRD patterns. This may be ascribed to the high entropy effect. For these alloys, high entropy of mixing (ǻS mix) caused by multi-principal elements significantly lower ǻG mix, which will make random solid solution easily form and more stable than intermetallic compounds or other ordered phases during solidification.The magnified image of (110) for BCC reflections is shown in Fig. 1(b). It is noticed that the (110) peak shifts towards lower 2ș as the Al contents increase, which indicates that the Al addition can cause the decrease of lattice parameters of NbTiVTaAl x alloys. Obviously, Al element has similar atomic radius to three elements of Nb, Ti and Ta, but Al has a distinctly larger atomic radius than V (see Table 1), which will affect the extent of lattice distortion and change the lattice constants of alloys.Table1. The crystal structure, atomic radius (r), melting temperature (T m) and density (ȡ) of high purity Nb, Ti, V, Ta and Al metals. The investigated crystal structure and the calculated melting temperature for the NTiVTaAl x alloy series are also given here.Al0Al0.25Al0.5Metal NbAlAl1.0TiVTaCrystal structure BCC HCP BCC BCC FCC BCC BCC BCC BCCr (pm) 147 146 135 147 143 ʊʊʊʊT m (K) 2750 194622023293933.525482453 23682225Fig. 2 SEM backscatter images of the (a) Al0, (b) Al0.25, (c) Al0.5and (d) Al1.0 alloys.Figure 2 shows the microstructures of NbTiVTaAl x alloys. It can be seen that the microstructure of Al0 alloy consists of equiaxial dendritic-like grains, and Al0.25, Al0.5, and Al1.0 alloys exhibit typical cast295 X. Yang et al. / P rocedia Engineering 36 ( 2012 )292 – 298296X. Yang et al. / P rocedia Engineering 36 ( 2012 )292 – 298Fig. 3 (a) The curves of ȍ and į as a function of Al contents for NbTiVTaAl x (x=0, 0.25, 0.5and 1) alloys; (b) the relation between VEC and Al contents of alloys.Fig. 4 (a) The compressive engineering stress-strain curves of NbTiVTaAl x (x = 0, 0.25, 0.5 and 1.0) alloys; (b) compressive test results for these alloys.Figure 4(a) shows the compressive engineering stress-strain curves of room temperature test for NbTiVTaAl x alloys. All alloys exhibit high yield compressive strength and good plastic deformation. The yield strength of Al0, Al0.25, Al0.5 and Al1.0 are 1092 MPa, 1330 MPa, 1012 MPa and 991 MPa, respectively. After yielding, the strength of alloys increase continuously and the samples of alloys do not break under about 50% compressive strain. The relations between yield strength, elastic modulus and Al contents for studied alloys are shown in Fig. 4(b). It can be seen that when the Al content increases to x = 0.25, the maximum yield strength increase to 1330 MPa; while the further addition of Al element can make yield strength decrease. This variation tendency of yield strength is similar to that of atomic size difference. Moreover, the elastic modulus of studied alloys changes slightly with the Al addition. The high yield strength of these alloys likely is attributed to solid solution strengthening.For NbTiVTaAl x alloys with single BCC structure, each atom can be expected as a solute atom and it can randomly occupy the crystal lattice site of alloy. Nevertheless these solute atoms with different sizes and properties can interact with each other and elastically distort the crystal lattice, which induce the297X . Yang et al. / P rocedia Engineering 36 ( 2012 ) 292 – 298 formation of local elastic stress field. The interactions between these local elastic stress fields and the stress field of dislocations in alloy will hinder dislocation movements, and cause the increase of strength. In general, the relation between the strengthening ('ı) and solute concentration (c) is expressed as [16]:n c V 'v (4) Here n § 0.5. Compared with the traditional crystal materials, the concentration of solute for NbTiVTaAl x HEAs is extremely high, so these alloys exhibit high compressive strength. On the other hand, the strengthening caused by atomic size mismatch will increase with the increase of atomic size difference. In NbTiVTaAl x HEAs, the atomic size difference reach a maximum as x = 0.25, the corresponding compressive yield strength of Al0.25 alloy also is the highest, which implies that the effect of atomic size mismatch on the strength is very notable in NbTiVTaAl x HEAs.Figure 5 shows the morphologies of the fractographs of the deformed sample for Al0 alloy. The lateral surface of the deformed sample under 50% compressive strains is shown in Fig. 5(a). It can be seen that the sample with the barrel-like form does not fracture after compressive deformation, but some surface cracks, marked by the arrows, are observed. Besides, the sample exhibits slightly nonuniform deformations, which may be ascribed to inhomogeneous microstructures. As a contrast, the secondary electron image and backscatter electron images of the deformed sample with 30% compressive strains are shown in Fig. 5 (b) and (c), respectively, no cracks can be observed in both surface and insider. Deformed grains that were elongated in the radial direction can be seen in Fig. 5 (c). The similar morphologies have also been observed in Al0.25, Al0.5 and Al1.0 alloys.Fig. 5 The morphologies of the fractographs of the deformed sample for Al0 alloy. (a) The lateral surface of the deformed sample under 50% compressive strains; the secondary electron image and backscatter electron images of the deformed sample with 30% compressive strains are shown in (b) and (c), respectively.4.ConclusionsA new series of NbTiVTaAl x high entropy alloys have been successfully prepared, which all have simple phase structures and exhibit obvious dendrite structures. The phase formation rule of them has298X. Yang et al. / P rocedia Engineering 36 ( 2012 )292 – 298been discussed, based on the parameters of ȍ, į and VEC. It is concluded that these alloys possess excellent BCC solid-solution formation ability. All alloys have high compressive yield strength and ductility (no fracture under 50% strains), which should be attributed to solid solution strengthening. AcknowledgementsThe authors would like to acknowledge financial support by the National Natural Science Foundation of China (NNSFC, No.50971019).References[1] Yeh J W, Chen S K, Lin S J, Gan J Y, Chin T S, Shun T T, et al. Nanostructured high-entropy alloys with multiple principalelements: Novel alloy design concepts and outcomes. Adv. Eng. Mater. 2004; 6(5): 299-303.[2] Zhou Y J, Zhang Y, Wang Y L, Chen G L. Microstructure and compressive properties of multicomponentAl x(TiVCrMnFeCoNiCu)100-x high-entropy alloys . Mater. Sci. Eng. A 2007; 454-455: 260-265.[3] Zhang Y, Zhou Y J, Lin J P, Chen G L, Liaw P K. Solid-solution phase formation rules for multi-component alloys. Adv.Eng. Mater. 2008; 10(6): 534-538.[4] Zhang Y, Chen G L, Gan C L. Phase Change and Mechanical Behaviors of Ti x CoCrFeNiCu1-y Al y High Entropy Alloys.Journal of ASTM International 2010; 7(5): 1-8.[5] Zhou Y J, Zhang Y, Wang Y L, Chen G L. Solid solution alloys of AlCoCrFeNiTi x with excellent room-temperaturemechanical properties. Appl. Phys. Lett. 2007; 90(18): 181904.[6] Chuang M H, Tsai M H, Wang W R, Lin S J, Yeh J W. Microstructure and wear behavior of Al x Co1.5CrFeNi1.5Ti y high-entropy alloys. Acta Mater 2011; 59(16): 6308-6317.[7] Wen L H, Kou H C, Li J S, Chang H, Xue X Y, Zhou L. Effect of aging temperature on microstructure and properties ofAlCoCrCuFeNi high-entropy alloy. Intermetallics 2009; 17(4): 266-269.[8] Tsai C W, Tsai M H, Yeh J W, Yang C C. Effect of temperature on mechanical properties of Al0.5CoCrCuFeNi wroughtalloy. J. Alloys Compd. 2010; 490(1-2): 160-165.[9] Zhu J M, Fu H M, Zhang H F, Wang A M, Li H, Hu Z Q. Microstructures and compressive properties of multicomponentAlCoCrFeNiMo x alloys [J]. Mater. Sci. Eng. A 2010; 527(26): 6975-6979.[10] Tong C J, Chen M R, Yeh J W, Lin S J, Chen S K, Shun T T, rt al. Mechanical performance of the Al x CoCrCuFeNi high-entropy alloy system with multiprincipal elements. Metall. Mater. Trans. A 2005; 36(5): 1263-1271.[11] Senkov O N, Wilks G B, Scott J M, Miracle D B. Mechanical properties of Nb25Mo25Ta25W25 andV20Nb20Mo20Ta20W20 refractory high entropy alloys. Intermetallics 2011; 19(5): 698-706.[12] Senkov O N, Wilks G B, Miracle D B, Chuang C P, Liaw P K. Refractory high-entropy alloys]. Intermetallics 2010; 18(9):1758-1765.[13] Yang X, Zhang Y. Prediction of High-entropy Stabilized Solid-solution in Multi-component Alloys. Mater. Chem. Phys.2011; in press.[14] Kittel C. Introduction to solid state physics. New York: Wiley. 1996.[15] Guo S, Ng C, Lu J, Liu C T. Effect of valence electron concentration on stability of fcc or bcc phase in high entropy alloys.J. Appl. Phys. 2011; 109(10): 103505-5.[16] Qiao J W, Ma S G, Huang E W, Chuang C P, Liaw P K, Zhang Y. Microstructural Characteristics and MechanicalBehaviors of AlCoCrFeNi High-Entropy Alloys at Ambient and Cryogenic Temperatures. Mater. Sci. Forum 2011; 688: 419-425.。