美国环保署农药地下水风险评估模型
应用美国国家环境保护署吸入风险模型评估职业危害的研究
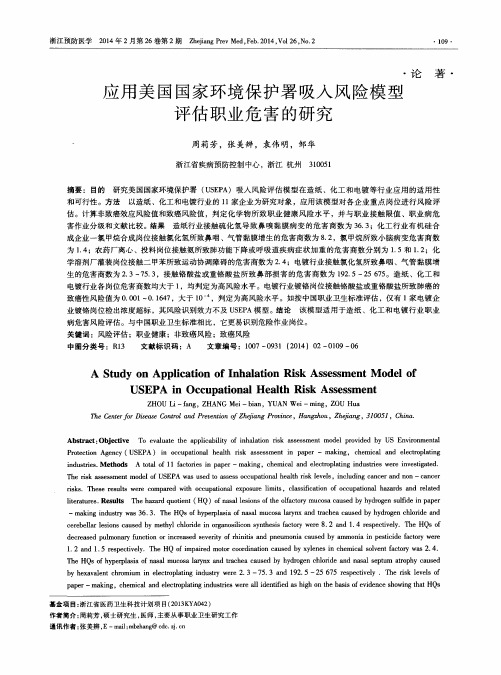
A b s t r a c t : Ob j e c t i v e T o e v a l u a t e t h e a p p l i c a b i l i t y o f i n h la a t i o n i r s k a s s e s s m e n t mo d e l p r o v i d e d b y U S E n v i r o n me n t l a P r o t e c t i o n A g e n c y( U S E P A) i n o c c u p a t i o n a l h e a l t h i r s k a s s e s s m e n t i n p a p e r— m a k i n g ,c h e m i c l a a n d e l e c t r o p l a t i n g
周莉 芳 ,张 美辨 ,袁 伟 明 ,邹华
浙江省疾 病预防控制 中心 ,浙江 杭州 摘要 :目的 3 1 0 0 5 1
研究美 国国家环境保 护署 ( U S E P A)吸入风险评 估模 型在造 纸 、化工和 电镀等行 业应 用的适用 性 以造纸 、化工 和电镀 行业 的 1 1家企业为研究对象 ,应用该模 型对各 企业 重点 岗位进行风 险评 造纸行业接触硫化 氢导致 鼻嗅黏膜 病变 的危害 商数为 3 6 . 3 ;化 工行业 有机硅合
i n d u s t i r e s . Me t h o d s A t o t a l o f 1 1 f a c t o i r e s i n p a p e r—ma k i n g,c h e mi c l a a n d e l e c t r o p l a t i n g i n d u s t ie r s we r e i n v e s t i g a t e d . T h e r i s k a s s e s s me n t mo d e l o f U S E P A w a s u s e d t o a s s e s s o c c u p a t i o n a l h e lt a h r i s k l e v e l s .i n c l u d i n g c a n c e r a n d n o n—c a n c e r i r s k s .T h e s e r e s u l t s we r e c o mp a r e d w i t h o c c u p a t i o n a l e x p o s u r e l i mi t s ,c l a s s i i f c a t i o n o f o c c u p a t i o n l a h a z a r d s a n d r e l a t e d
美国地下水和饮用水井中挥发性有机物分析
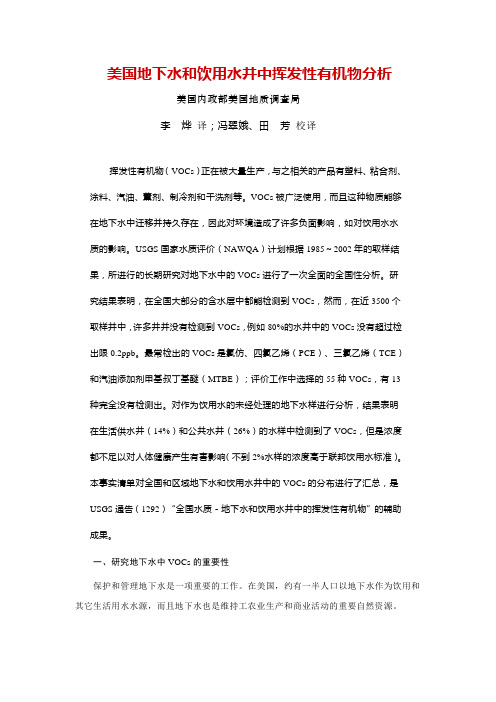
美国地下水和饮用水井中挥发性有机物分析美国内政部美国地质调查局李烨译;冯翠娥、田芳校译挥发性有机物(VOCs)正在被大量生产,与之相关的产品有塑料、粘合剂、涂料、汽油、薰剂、制冷剂和干洗剂等。
VOCs被广泛使用,而且这种物质能够在地下水中迁移并持久存在,因此对环境造成了许多负面影响,如对饮用水水质的影响。
USGS国家水质评价(NAWQA)计划根据1985~2002年的取样结果,所进行的长期研究对地下水中的VOCs进行了一次全面的全国性分析。
研究结果表明,在全国大部分的含水层中都能检测到VOCs,然而,在近3500个取样井中,许多井并没有检测到VOCs,例如80%的水井中的VOCs没有超过检出限0.2ppb。
最常检出的VOCs是氯仿、四氯乙烯(PCE)、三氯乙烯(TCE)和汽油添加剂甲基叔丁基醚(MTBE);评价工作中选择的55种VOCs,有13种完全没有检测出。
对作为饮用水的未经处理的地下水样进行分析,结果表明在生活供水井(14%)和公共水井(26%)的水样中检测到了VOCs,但是浓度都不足以对人体健康产生有害影响(不到2%水样的浓度高于联邦饮用水标准)。
本事实清单对全国和区域地下水和饮用水井中的VOCs的分布进行了汇总,是USGS通告(1292)“全国水质-地下水和饮用水井中的挥发性有机物”的辅助成果。
一、研究地下水中VOCs的重要性保护和管理地下水是一项重要的工作。
在美国,约有一半人口以地下水作为饮用和其它生活用水水源,而且地下水也是维持工农业生产和商业活动的重要自然资源。
VOCs是一类重要的环境污染物,由于其广泛和长期的使用,因而需要监测和管理地下水中的这类物质。
许多VOCs可以在地下水中运移和滞留,其中一些已经确定或怀疑对人类有致癌作用,美国环保局(USEPA)对公共水系统中的VOCs浓度进行了控制。
VOCs可以释放到环境中,通过不同的污染源和途径到达地下水,包括汽油发动机排气、工业废气、储油罐泄漏、垃圾填埋、城市废水入渗、化粪池和水井的注入等。
地下水污染风险评价及方法
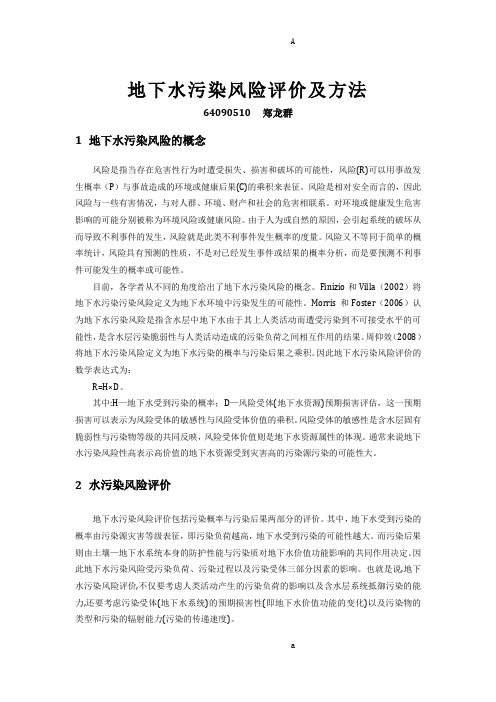
地下水污染风险评价及方法64090510 郑龙群1 地下水污染风险的概念风险是指当存在危害性行为时遭受损失、损害和破坏的可能性,风险(R)可以用事故发生概率(P)与事故造成的环境或健康后果(C)的乘积来表征。
风险是相对安全而言的,因此风险与一些有害情况,与对人群、环境、财产和社会的危害相联系。
对环境或健康发生危害影响的可能分别被称为环境风险或健康风险。
由于人为或自然的原因,会引起系统的破坏从而导致不利事件的发生,风险就是此类不利事件发生概率的度量。
风险又不等同于简单的概率统计,风险具有预测的性质,不是对已经发生事件或结果的概率分析,而是要预测不利事件可能发生的概率或可能性。
目前,各学者从不同的角度给出了地下水污染风险的概念。
Finizio和Villa(2002)将地下水污染污染风险定义为地下水环境中污染发生的可能性。
Morris和Foster(2006)认为地下水污染风险是指含水层中地下水由于其上人类活动而遭受污染到不可接受水平的可能性,是含水层污染脆弱性与人类活动造成的污染负荷之间相互作用的结果。
周仰效(2008)将地下水污染风险定义为地下水污染的概率与污染后果之乘积。
因此地下水污染风险评价的数学表达式为:R=H×D。
其中:H—地下水受到污染的概率;D—风险受体(地下水资源)预期损害评估,这一预期损害可以表示为风险受体的敏感性与风险受体价值的乘积。
风险受体的敏感性是含水层固有脆弱性与污染物等级的共同反映,风险受体价值则是地下水资源属性的体现。
通常来说地下水污染风险性高表示高价值的地下水资源受到灾害高的污染源污染的可能性大。
2 水污染风险评价地下水污染风险评价包括污染概率与污染后果两部分的评价。
其中,地下水受到污染的概率由污染源灾害等级表征,即污染负荷越高,地下水受到污染的可能性越大。
而污染后果则由土壤—地下水系统本身的防护性能与污染质对地下水价值功能影响的共同作用决定。
因此地下水污染风险受污染负荷、污染过程以及污染受体三部分因素的影响。
水产品风险评估农药地表水
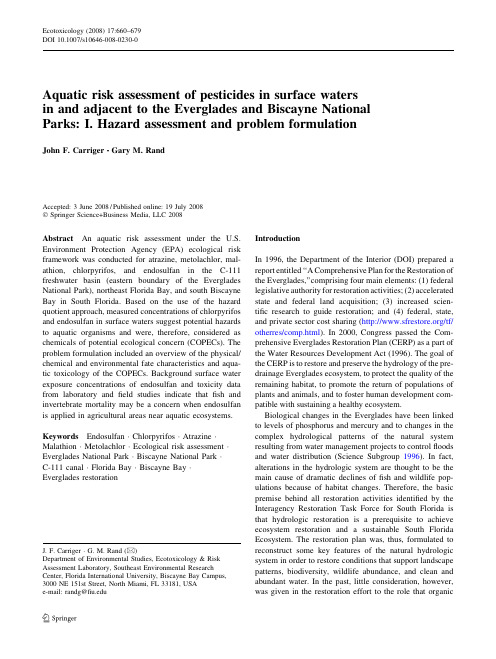
Aquatic risk assessment of pesticides in surface waters in and adjacent to the Everglades and Biscayne National Parks:I.Hazard assessment and problem formulationJohn F.Carriger ÆGary M.RandAccepted:3June 2008/Published online:19July 2008ÓSpringer Science+Business Media,LLC 2008Abstract An aquatic risk assessment under the U.S.Environment Protection Agency (EPA)ecological risk framework was conducted for atrazine,metolachlor,mal-athion,chlorpyrifos,and endosulfan in the C-111freshwater basin (eastern boundary of the Everglades National Park),northeast Florida Bay,and south Biscayne Bay in South Florida.Based on the use of the hazard quotient approach,measured concentrations of chlorpyrifos and endosulfan in surface waters suggest potential hazards to aquatic organisms and were,therefore,considered as chemicals of potential ecological concern (COPECs).The problem formulation included an overview of the physical/chemical and environmental fate characteristics and aqua-tic toxicology of the COPECs.Background surface water exposure concentrations of endosulfan and toxicity data from laboratory and field studies indicate that fish and invertebrate mortality may be a concern when endosulfan is applied in agricultural areas near aquatic ecosystems.Keywords Endosulfan ÁChlorpyrifos ÁAtrazine ÁMalathion ÁMetolachlor ÁEcological risk assessment ÁEverglades National Park ÁBiscayne National Park ÁC-111canal ÁFlorida Bay ÁBiscayne Bay ÁEverglades restorationIntroductionIn 1996,the Department of the Interior (DOI)prepared a report entitled ‘‘A Comprehensive Plan for the Restoration of the Everglades,’’comprising four main elements:(1)federal legislative authority for restoration activities;(2)accelerated state and federal land acquisition;(3)increased scien-tific research to guide restoration;and (4)federal,state,and private sector cost sharing (/tf/otherres/comp.html ).In 2000,Congress passed the Com-prehensive Everglades Restoration Plan (CERP)as a part of the Water Resources Development Act (1996).The goal of the CERP is to restore and preserve the hydrology of the pre-drainage Everglades ecosystem,to protect the quality of the remaining habitat,to promote the return of populations of plants and animals,and to foster human development com-patible with sustaining a healthy ecosystem.Biological changes in the Everglades have been linked to levels of phosphorus and mercury and to changes in the complex hydrological patterns of the natural system resulting from water management projects to control floods and water distribution (Science Subgroup 1996).In fact,alterations in the hydrologic system are thought to be the main cause of dramatic declines of fish and wildlife pop-ulations because of habitat changes.Therefore,the basic premise behind all restoration activities identified by the Interagency Restoration Task Force for South Florida is that hydrologic restoration is a prerequisite to achieve ecosystem restoration and a sustainable South Florida Ecosystem.The restoration plan was,thus,formulated to reconstruct some key features of the natural hydrologic system in order to restore conditions that support landscape patterns,biodiversity,wildlife abundance,and clean and abundant water.In the past,little consideration,however,was given in the restoration effort to the role that organicJ.F.Carriger ÁG.M.Rand (&)Department of Environmental Studies,Ecotoxicology &Risk Assessment Laboratory,Southeast Environmental Research Center,Florida International University,Biscayne Bay Campus,3000NE 151st Street,North Miami,FL 33181,USA e-mail:randg@fiEcotoxicology (2008)17:660–679DOI 10.1007/s10646-008-0230-0pesticides and other contaminants play in the structure and function of ecosystems,although this was clearly recommended by the Science Subgroup(1996)in all physiographic regions that comprise South Florida.This was further supported at a workshop entitled‘‘Linking Ecotoxicity and Risk Management to Sustainable Resto-ration of South Florida Ecosystems,’’which recommended screening-level ecological risk assessments with retro-spective and prospective diagnostic studies(LaPoint et al. 1998).It is evident that water quantity rather than water quality issues have dominated the South Florida restoration plan-ning(Scott et al.2002).However,it is also evident that agriculture represents a major land use in South Florida and pesticide use presents a potential risk,especially to aquatic organisms.Based on a hazard ranking of pesticides by the National Oceanic and Atmospheric Administration (NOAA),the top three estuarine drainage areas at risk in the U.S.were in Florida-Rookery Bay,Biscayne Bay,and Tampa Bay(Pait et al.1992).The subtropical climate,long crop-growing season,application frequency,and multitude of uses(e.g.,mosquito and termite control,golf courses, and landscape management)renders pesticides particularly hazardous in South Florida ecosystems.The Canal111(Aerojet Canal or C-111)freshwater basin(Fig.1)is a buffer zone that separates the wetlands of the Everglades National Park(ENP)from highly produc-tive subtropical agricultural lands and urban development to the east,and while considerable attention and resources have been allocated to altering the hydrology of this landscape,little effort has focused on understanding water quality issues that may arise from land use practices.Thus far,analytical monitoring programs have detected the presence of organic pesticides in the surface water of either the lower C-111freshwater canal basin and/or its confluent estuaries/saltwater systems.For example,the South Florida Water Management District(SFWMD)began monitoring pesticides in the water and sediment of South Florida canals in the mid-1980s(Pfeuffer1985,1991).Sediment and water analyses by SFWMD indicates that atrazine, ametryn,bromacil,simazine,diuron,alpha(a)-endosulfan, beta(b)-endosulfan,endosulfan sulfate,ethion,hexazi-none,and norflurazon were the most frequently detected pesticides in surface water and DDE,DDD,ametryn, atrazine,dicofol,diquat,and endosulfan sulfate were the most frequently detected pesticides in sediment samples between1991and1995(Miles and Pfeuffer1997).Several of the sampling sites were located in the Everglades Agricultural Area(EAA)and others in the Homestead Agricultural Area(HAA)adjacent to the Everglades National Park.Detectable endosulfan residues(a and b isomers,and sulfate metabolite)in the C-111(at S-178) were consistently present in the surface water from1991to 1995,and occasionally exceeded the Florida Department of Environmental Protection(FDEP)and U.S.Environment Protection Agency(EPA)water quality criteria(Miles and Pfeuffer1997).Surface water samples collected indepen-dently by the NOAA from the southern SFWMD sampling sites confirmed thesefindings(Scott et al.1994).Residues of endosulfan sulfate were also consistently found by the SFWMD in sediment samples at S-178in the C-111,while the a and b isomers were occasionally found.All three endosulfan residues were also found in sediment samples from structures S-177and S-18C in the C-111.The SFWMD summarized endosulfan sulfate residues in S-178 water and found that the FDEP water quality criteria were exceeded11times from1996to2000(two samples were from NOAA;R.Pfeuffer,personal communication).In a SFWMD monitoring program of South Florida canals from 1992to2001,the most common pesticides in surface water were the herbicides atrazine and ametryn,while DDE and DDD were the most frequently detected pesticides in sed-iment samples(Pfeuffer and Rand2004).The U.S.EPA,in 1995,also monitored contaminants in surface water,sedi-ment,and biota in the C-111and creeks of northeast Florida Bay(Goodman et al.1999).Endosulfan residues were detected in sediments of the C-111,and in sediments of Shell and Trout Creeks(in northeast Florida Bay). Organochlorine contaminants occurred at low concentra-tions in sediments of canals and creeks,and PCBs and PAHs were also at low concentrations but higher in the C-111than in creeks.At most sampling sites for water and sediment,more than one pesticide was detected in each sample.The NOAA conducted sediment toxicity tests and a contaminant monitoring study of the C-111and Florida Bay from1993to1997,but it did not evaluate cause (exposure)–effect(toxicity)relationships for contaminants (Scott et al.2002).It did however;indicate the presence of low levels of endosulfan(total),atrazine,chlorpyrifos,and chlorothalonil in surface waters of canals adjacent to agricultural areas that drain into the C-111and in northeast Florida Bay waters.Florida Bay waters occasionally exceeded the U.S.EPA marine water quality criterion (WQC)for endosulfan.Waters from canal sites also con-tained detectable concentrations of endosulfan that sporadically exceeded U.S.EPA fresh WQC.Detectable endosulfan(total)residues were also found in sediment and oysters,while chlorpyrifos was detected infish tissue. Toxicity tests with in-place sediment and copepods and bivalves indicated potential adverse effects,but the caus-ative agent(s)was not determined.The highest concen-tration of endosulfan(total)reached477ng/l,and10%of the samples from canal sites exceeded the U.S.EPA chronic freshwater WQC(56ng/l)(47%of the canal sites had detectable levels of endosulfan).The U.S.EPA chronicHazard assessment and problem formulation661marine WQC (8.7ng/l)for endosulfan was exceeded at 2%of the sites in Florida Bay,while 39%of bay sites had detectable endosulfan concentrations.The highest per-centage (40%)of water quality violations for endosulfan,based on U.S.EPA standards,was detected in samples from S-178.The NOAA,in 1999–2000,also found that endo-sulfan concentrations were highest (mean dry season concentration *300ng/l)in the C-111E (Fulton et al.2004).Data from the NOAA’s National Status and Trends (NS&T)Program Mussel Watch Project further indicated that the mean annual concentrations of endosulfan (II,b -isomer)residue in tissues (oysters)sampled from Joe Bay (in northeast Florida Bay)were higher than the NS&T 85th percentile (i.e.,it is in the highest 15%of the data set,with over 280sites nationwide)(Cantillo et al.1999).Recently,Harman-Fetcho et al.(2005)found that atrazine,metola-chlor,chlorothalonil,chlorpyrifos,and total endosulfan (a -+b -+endosulfan sulfate)were the most frequently detected pesticides in water samples from South Florida canals based on a 2-year study from 2002to 2004.Atrazine had the highest concentration (108ng/l),followed by endosulfan (total;98ng/l),metolachlor (86ng/l),chlor-pyrifos (58ng/l),and chlorothalonil (14ng/l).In addition,Carriger et al.(2006)identified DDT,DDD,DDE,chlor-dane,and endosulfan (total)as chemicals of potential ecological concern (COPECs)in the sediment of South Florida canals based on the exceedence of sediment quality criteria in a two-tier sediment probabilistic risk assessment.Endosulfan had the highest potential risk (chronic)to arthropods at S-178on the C-111system.To address the concerns about pesticides in the C-111basin,the National Park Service (U.S.DOI)requested that an aquatic probabilistic screening level ecological risk assess-ment (SERA)be conducted.It focuses on the risk of adverse effects from pesticide exposures in surface water on aquatic organisms in a freshwater canal (C-111system)and its confluent estuarine/saltwater systems (northeastFloridaFig.1Land use and drainage canals in the C-111flood control basin in southeast Florida662J.F.Carriger,G.M.RandBay–Joe Bay,Long Sound,Highway Creek;Card Sound in South Biscayne Bay).To date,this is the only site-specific SERA conducted as part of the Everglades restoration effort, and it is being‘‘exposure-driven’’(Suter1993).Presently, there is little evidence that documented pesticide exposures in surface water are eliciting adverse biological effects in aquatic receptors in these systems or on their potential risk. This SERA applied the ecological risk assessment(ERA) framework under the current U.S.EPA guidelines(U.S.EPA 1998)and it addresses the likelihood and ecological signif-icance of the potential effects of surface water exposures to the herbicides atrazine and metolachlor,and the insecticides malathion,chlorpyrifos,and endosulfan obtained from monitoring programs from the U.S.Geological Survey (USGS),the SFWMD,and the NOAA.These pesticides were detected by the various monitoring programs with the highest frequency in the C-111system and northeast Florida Bay and south Biscayne Bay.There have been chemical-specific aquatic ecological risk assessments conducted thus far on atrazine(Solomon et al.1996;Giddings et al.2000), chlorpyrifos(Giesy et al.1999;Hall and Anderson2003), and endosulfan(U.S.EPA2002a,2007).This SERA was intended to assess the potential risk of these pesticides in surface water,and not to determine the actual causes of any declines in the populations of native invertebrates,fish,or plants that may be prevalent in the above ecosystems.Since each pesticide is not used in iso-lation to control pests and may co-occur;potential risks associated with the effects of joint actions of these pesticides were also considered.Assessment of the potential impact of thefive pesticides on aquatic communities acknowledges that sea level rise,hurricanes,development,historical water management activities,and salinity changes may have altered habitats and affected populations.Furthermore,other chemical,non-chemical stressors in water and/or sediment are not being considered and may likely also contribute to adverse impacts in the study areas.Because the SERA con-tained a large database,the results are presented in two papers.This paper discusses the general ecological risk assessment methods and the results of Tier1—hazard assessment and problem formulation for the pesticides in the C-111system and northeast Florida Bay and south Biscayne Bay.The subsequent paper discusses the methods and results of Tier2—probabilistic analyses of the chemicals(i.e., pesticides)of potential ecological concern(COPECs)in the C-111,northeast Florida Bay,and south Biscayne Bay. Study area descriptionThe C-111basin(100square miles)is located in southeastern Dade County,Florida,adjacent to the eastern boundary of the Everglades National Park(ENP)(Fig.1).It includes lands that lie to the southeast boundary of the East Everglades and west of the coastal basins and includes the Frog Pond(i.e., agricultural area).It,thus,drains the agricultural areas of South Dade County.South of Homestead,the C-111is joined by the C-111E and it then moves south and southeastward to cross marl marsh,whichflows into Manatee Bay at the head of Barnes Sound,a semi-enclosed lagoonal estuary of south Biscayne Bay.Surface water runoff from the C-111basin represents an important source of freshwaterflow into the ENP and the estuarine ecosystems of northeast Florida Bay through Taylor Slough and south Biscayne Bay through the S-197(in C-111)into Manatee Bay.Low tidal range and long flushing times make Manatee Bay particularly vulnerable to the effects of large freshwater inflows.Such pulses of fresh-water persist for long periods of time and move within and between the shallow estuaries that make up South Biscayne Bay and its associated sounds(Fatt and Wang1987).Unfor-tunately,low tidal range and longflushing times also make Manatee Bay and Barnes Sound vulnerable to hyper-saline conditions during periods of reduced freshwaterflow.During the dry season,saltwater moves inland and the Western shore of south Biscayne Bay frequently experiences high salinities (Wang et al.1978).Northeast Florida Bay includes the downstream freshwater marshes and estuarine systems that extend from the southern edge of Barnes Sound on the east to Madeira Bay on the west,and include Little Madeira Bay,Joe Bay,Highway Creek,and Long Sound.Florida Bay is a triangular-shaped estuary composed of basins,banks,and islands that lie between the southern tip of the Florida mainland and the Florida Keys.It has a shallow depth(mean1m)that is perfect for light penetration and the sustainability of seagrass beds,which are a dominant habitat and a source of productivity in the Bay.The salinity of the Bay can rise to twice that of seawater as a result of the long residence time and shallow depth(McIvor et al.1997).The sediments of the Bay are composed of carbonate mud,which sorb inorganic phosphorus from water(de Kanel and Morse 1978).The Bay was healthy until the mid-1980s,when cat-ches of pink shrimp(Penaeus duorarum)declined(Browder et al.1999)and the mass mortality of turtle grass(Thalassia testudinum)began(Robblee et al.1991).By the1990s,the Bay ecosystem appeared to shift from a clear water system dominated by benthic primary production to a turbid system dominated by algal blooms and resuspended sediment. Although there has been no dramatic decrease in totalfish abundance,there has been a shift in species composition as a result of seagrass habitat loss and algal blooms(Davis and Ogden1997).Fish that consume algae,such as the bay anchovy,are increasing.When large volumes of freshwater are discharged from the C-111canal,this water tends to move into coastal waters and estuaries that can create local problems due to the transfer of freshwater,contaminants,and suspendedHazard assessment and problem formulation663materials from urban and agricultural land.Freshwater inflow from the C-111and surface runoff further transports nutrients and detritus from adjacent marshes and uplands into south Biscayne Bay and northeast Florida Bay.Sam-pling sites for water monitoring programs in the C-111, Florida Bay,and Biscayne Bay are labeled in Fig.1.The Miami River is an additional source of contaminants into Biscayne Bay(Long et al.2002).In the1960s,the C-111area was channelized as part of the comprehensive Central and Southern Florida(C&SF)Flood Control Project.At that time,this area was envisioned as urban development,but by the1980s,it was clear that the C-111 drainage system,which had undergone several revisions,had significantly contributed to a decline in the natural resources of the ENP.The current revision of the system,authorized in 1996,promises to restore some of the natural hydropatterns to Taylor Slough,the eastern panhandle areas of the ENP,and improve estuarine conditions in Florida Bay(project description can be found at / dp/mwdenp-c111/index.htm).MethodsThe SERA consisted of thefirst three phases of the U.S.EPA ERA framework(U.S.EPA1998):problem formulation, analysis,and risk characterization.Problem formulation defined the problem and the plan for analyzing and charac-terizing the risk.Data on stressor characteristics,ecosystems at risk,ecological effects,ecosystem(s),and receptor(s) characteristics were synthesized for this phase.From this data,assessment(i.e.,what we are trying to protect)and measurement(i.e.,tools used to measure effects on assess-ment endpoints)and a conceptual model were developed to prepare the analysis plan(i.e.,where risk hypotheses were evaluated).The conceptual model at the completion of problem formulation uses information on the ecosystems at risk,stressor characteristics,biological effects,and the relationship between endpoints to define exposure and effects scenarios.The objective of the conceptual model is to formulate hypotheses to determine how the pesticide stressors may affect ecosystems that are exposed.The second phase of the SERA was risk analysis and it characterized and examined two major components of risk; exposure and effects.Risk characterization was thefinal phase.This provided potential risk estimates to the eco-logical entities listed as assessment endpoints based on the occurrence and magnitude of exposures and the severity of adverse effects resulting from such exposures.Analyses (exposure and effects characterization)and risk character-ization are discussed in the follow-up paper.A tiered ecological risk characterization approach was suggested by the ARAMDG(SETAC1994)and endorsed by the U.S.EPA(ECOFRAM1999)that uses a stepwise approach progressing from the simple Tier1hazard quo-tient(HQ)approach to a more complex Tier2probabilistic risk assessment(PRA).A two-tier approach was used in the SERA.In Tier1,the HQ approach wasfirst used with a screening benchmark,followed by problem formulation. Screening benchmarks are concentrations of chemicals that are believed to constitute thresholds for the potential toxic effects of some ecological receptor exposed to a chemical in some medium(Suter and Tsao1996).The U.S.EPA Water Quality Criteria(WQC)and Sediment Quality Cri-teria(SQC)are commonly used as screening benchmarks because the exceedence of one of these values constitutes cause for concern.In the SERA,actual measured envi-ronmental concentrations(AECs)of the pesticides in surface waters were compared to the U.S.EPA WQC values that were available(i.e.,endosulfan,chlorpyrifos,mala-thion,and atrazine)to obtain an HQ.No WQC were available for metolachlor.Therefore,the AECs of met-olachlor were compared to the response concentration for the most sensitive species in a toxicity test(i.e.,from the lowest LC50/EC50,lowest NOEC from chronic tests)to obtain an HQ.AECs in surface waters were obtained from monitoring programs from state(SFWMD)and federal(NOAA, USGS)agencies for1999–2000.Site numbers and the location of sampling sites where pesticide concentrations (atrazine,chlorpyrifos,endosulfan,malathion,and met-olachlor)were measured with land use characteristics are shown in Fig.1.The SFWMD did not measure for chlor-pyrifos,the NOAA did not measure for malathion,and the USGS did not measure for endosulfan.Monitoring data were available for11freshwater sites(S-175,S-176, S-332,Site A,S-177/site B,S-178/site C,S-18C/site E, Site W1,Site W2,Site E1,and Site E2)on or near the C-111and three estuarine sites(Joe Bay and Highway Creek in northeast Florida Bay and Card Sound in south Biscayne Bay).Sites in W1,W2,E1,E2,Highway Creek, and Joe Bay were located in the Everglades panhandle.When the quotient of the exposure concentration to the criteria value(or lowest acute toxicity value for metola-chlor)was greater than1,an adverse effect(i.e.,high hazard)was expected to occur.For endosulfan,there are separate freshwater and saltwater criteria for a-and b-endosulfan,but no criterion exists for endosulfan sulfate, a toxic oxidation metabolite.Since the endosulfan WQC was generated from aquatic toxicity studies with technical-grade endosulfan,each criterion is applicable to the sum-mation of the a-and b-isomers(U.S.EPA2002b).We were,therefore,conservative in Tier1and compared total endosulfan concentrations(i.e.,summation of concentra-tions of a and b isomers plus the endosulfan sulfate664J.F.Carriger,G.M.Randmetabolite)to the criterion to obtain an HQ.HQ excee-dences in Tier1were then used to focus on COPECs for problem formulation and Tier 2.Tier2,probabilistic risk assessment,characterizes risk by comparing the probability distributions of surface water exposure con-centrations with the probability distributions of species response data from laboratory toxicity studies.Results for Tier1—hazard assessment and problem formulation are discussed below.Results and discussionTier1—hazard assessmentSample sites,number of samples collected,and the fre-quency of detection for each pesticide in freshwater and estuarine sites are presented in Table1for the two-year period(1999–2000).Sites with exceedences of WQC for each pesticide are listed in Table2.The herbicide atrazine was the most frequently detected pesticide.It was detected in92%of the185freshwater samples in1999and100%of the106samples taken in2000.The highest detected con-centration of atrazine in freshwater was0.337l g/l(at S-18C/site E).It was detected in88%of the24estuarine samples in1999and81%of the26samples taken in2000. The highest detected concentration of atrazine in saltwater was0.104l g/l(at Joe Bay).Concentrations of atrazine did not exceed freshwater or marine WQC.Acute and chronic HQs were low and indicated no ecological hazard to fresh-or salt-water organisms.The other herbicide measured,metolachlor,was only detected in28%of the185freshwater samples in1999and 26%of the106samples taken in2000.It was not detected in24estuarine samples in1999and was only detected in 12%of the26samples taken in2000.The acute and chronic HQs for metolachlor in freshwater and saltwater were close to zero when the peak exposure concentrations of the herbicide were compared with the lowest toxicity values.The pesticide with the lowest number of detections was malathion.Malathion was found about4%of the time at freshwater sites and0%of the time at estuarine sites, respectively,in1999and2000.Atrazine,metolachlor,and malathion were not COPECs and,therefore,were not considered for Tier2single chemical probabilistic risk assessments.However,they were considered as potential co-joint(additive)stressors in Tier2when they were present at detectable concentrations.Chlorpyrifos was detected in48%of the89freshwater samples in1999and85%of the91samples taken in2000. It was detected in79%of the24estuarine samples in1999 and96%of the26samples taken in2000.The two highest concentrations for chlorpyrifos were found at S-177/site B at0.0234and0.0232l g/l,which were nearly four times higher than the next highest maximum concentration, which was measured at W2,where it was found86%of the time.The maximum concentration value for chlorpyrifos was found during the dry season in February1999.The only water quality violation for chlorpyrifos occurred in Joe Bay in1999.The acute and chronic HQs for freshwater indicated no potential hazard,but the acute HQs for estu-arine water indicated potential hazard.Although there was only one WQC violation for chlorpyrifos,it was considered as a COPEC because several AECs were just below WQC.Endosulfan was detected in45%of the173freshwater samples in1999and90%of the93samples taken in2000. It was detected in96%of the24estuarine samples in1999 and96%of the26samples taken in2000.Endosulfan concentrations were detected infrequently at S-176(1out of32),S-332(2out of32),and S-175(0out of26).The highest concentration of endosulfan was found at S-178/ site C in February2000,followed by S-177/site B,where concentrations peaked in the dry season of1999and2000. S-18C/site E had the third highest detected concentrations for endosulfan,which occurred in February1999and2000. E1,W1,and W2,which are downstream of S-177/site B and S-178/site C,had100%detections for endosulfan. Water quality violations for endosulfan were found in freshwater and estuarine sites.The majority of violations occurred at S-178/site C,a site closest to the Frog Pond agricultural area.Out of266 samples taken for analyses of endosulfan in the C-111 during1999and2000,7.5%violated freshwater WQC.Of the20water quality violations at S-178/site C,eight did not have detectable concentrations of a-endosulfan.However, the highest concentration(1.345l g/l)had the highest percentage concentration of a-endosulfan and the lowest percentage concentration of endosulfan sulfate.In general, concentrations of b-endosulfan were also low.Except for one sample,the majority of total endosulfan at S-178/site C sample violations was made up of endosulfan sulfate(72–100%of each sample).The fact that endosulfan sulfate is the major metabolite of endosulfan in aquatic systems supports other recent work(Laabs et al.2007;Shivara-maiah et al.2005).In estuarine sites,endosulfan water quality violations were found at all three sites sampled and,out of50samples taken at Highway Creek or Joe Bay,20%violated saltwater WQC for endosulfan.In these same sites,endosulfan sul-fate made up71–94%of the total endosulfan of samples with water quality violations.The acute and chronic HQs for total endosulfan in freshwater indicated potential haz-ards and the acute HQ for estuarine water also indicated potential hazards.Endosulfan generally had the highest measured concentrations in freshwater and estuarine sites at the end of the dry season for each year.Hazard assessment and problem formulation665。
城市环境污染的三种健康风险评价模型及比较
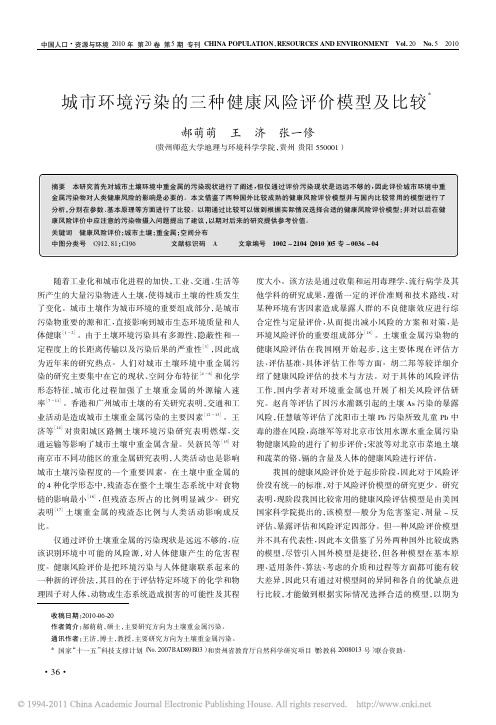
围内; 当 CDI / HCV > 1,说明污染场地具有潜在的健康风
险。
1. 3 HHRE( Human health risk evaluation)模型
HHRE 模型是由欧洲官方提供的,为解决规划带来的
土地利用对土壤重金属的影响和风险。该模型指潜在风
险源对人类与土壤重金属的接触( 暴露途径) 的影响,它
ence Dose) 值为衡量标准,暴露剂量率和参考剂量的关系 HQ = CDI / RfD,RfD 污染物的参考剂量,因此非致癌物质
的危害商( HQ) 计算公式如下:
HQ = C × IRoral × EForal × EDoral BW × AT × RfDoral
+ C × IRdermal × EFdermal × EDdermal BW × AT × RfDdermal
对于非致癌物质计算其危害商判定标准设定为112cleac???a???a???la??e???????a?????????模型clea模型是有英国环保署和环境食品与农村事务部以及苏格兰环境保护局联合开发是英国官方推荐用来进行污染场地评价以及获取土壤指导限值sgvs的模型35
中国人口·资源与环境 2010 年 第 20 卷 第 5 期 专刊 CHINA POPULATION,RESOURCES AND ENVIRONMENT Vol. 20 No. 5 2010
价。一级评价仅针对污染源点上方的暴露点,也即假定污
染物暴露的受体位置在污染点原位。评价所需的土壤、地
下水、大气、污染物特性等参数大量采用经验保守值。二
级评价针对 污 染 影 响 区 内 的 真 实 暴 露 点,相 比 于 一 级 评
价,二级评价在分析与污染源点异位的暴露点时,需要考
废弃农药厂土壤和地下水中有机磷农药的健康风险评价
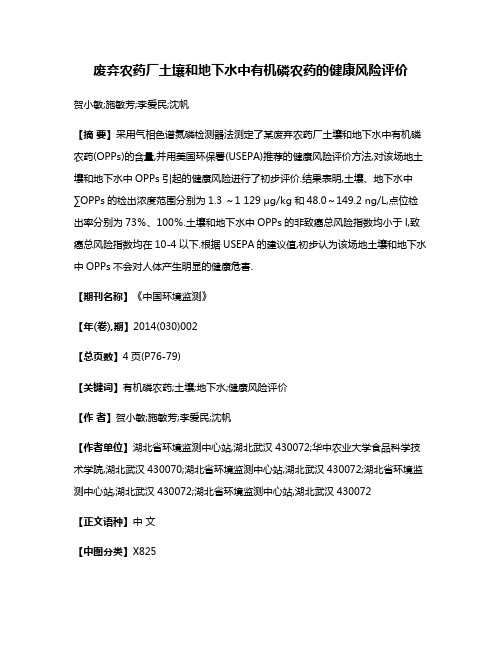
废弃农药厂土壤和地下水中有机磷农药的健康风险评价贺小敏;施敏芳;李爱民;沈帆【摘要】采用气相色谱氮磷检测器法测定了某废弃农药厂土壤和地下水中有机磷农药(OPPs)的含量,并用美国环保署(USEPA)推荐的健康风险评价方法,对该场地土壤和地下水中OPPs引起的健康风险进行了初步评价.结果表明,土壤、地下水中∑OPPs的检出浓度范围分别为1.3 ~1 129 μg/kg和48.0~149.2 ng/L,点位检出率分别为73%、100%.土壤和地下水中OPPs的非致癌总风险指数均小于l,致癌总风险指数均在10-4以下.根据USEPA的建议值,初步认为该场地土壤和地下水中OPPs不会对人体产生明显的健康危害.【期刊名称】《中国环境监测》【年(卷),期】2014(030)002【总页数】4页(P76-79)【关键词】有机磷农药;土壤;地下水;健康风险评价【作者】贺小敏;施敏芳;李爱民;沈帆【作者单位】湖北省环境监测中心站,湖北武汉430072;华中农业大学食品科学技术学院,湖北武汉430070;湖北省环境监测中心站,湖北武汉430072;湖北省环境监测中心站,湖北武汉430072;湖北省环境监测中心站,湖北武汉430072【正文语种】中文【中图分类】X825有机磷农药(OPPs)是目前中国农药市场份额最大、在农业活动中使用最广的一类杀虫剂。
由于OPPs具有一定的有效起始活性和残留活性, 因而对生态环境及人体健康可能构成潜在的风险与威胁。
OPPs中的马拉硫磷、乙基对硫磷等已被日本等国列入“环境激素黑名单”[1]。
中国68种环境优先污染物黑名单中,OPPs占5种,分别为敌敌畏、乐果、对硫磷、甲基对硫磷、敌百虫。
近年来, 许多城市正将一些污染较重的工业企业搬出人口密集城区。
拆迁后的原工业场地往往规划为商业或居住用地。
这些工业场地在长期的工业生产活动中往往受到不同程度污染, 为保护人体健康, 在转化使用功能前, 需要对场地土壤和地下水进行环境风险评价[2]。
美国的地下水水质监测
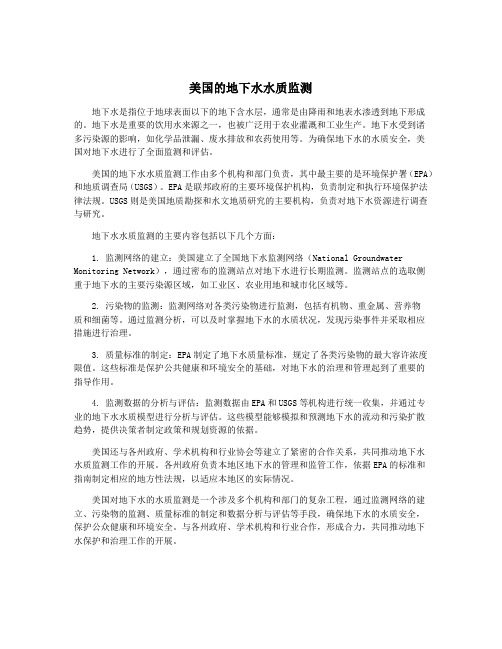
美国的地下水水质监测地下水是指位于地球表面以下的地下含水层,通常是由降雨和地表水渗透到地下形成的。
地下水是重要的饮用水来源之一,也被广泛用于农业灌溉和工业生产。
地下水受到诸多污染源的影响,如化学品泄漏、废水排放和农药使用等。
为确保地下水的水质安全,美国对地下水进行了全面监测和评估。
美国的地下水水质监测工作由多个机构和部门负责,其中最主要的是环境保护署(EPA)和地质调查局(USGS)。
EPA是联邦政府的主要环境保护机构,负责制定和执行环境保护法律法规。
USGS则是美国地质勘探和水文地质研究的主要机构,负责对地下水资源进行调查与研究。
地下水水质监测的主要内容包括以下几个方面:1. 监测网络的建立:美国建立了全国地下水监测网络(National Groundwater Monitoring Network),通过密布的监测站点对地下水进行长期监测。
监测站点的选取侧重于地下水的主要污染源区域,如工业区、农业用地和城市化区域等。
2. 污染物的监测:监测网络对各类污染物进行监测,包括有机物、重金属、营养物质和细菌等。
通过监测分析,可以及时掌握地下水的水质状况,发现污染事件并采取相应措施进行治理。
3. 质量标准的制定:EPA制定了地下水质量标准,规定了各类污染物的最大容许浓度限值。
这些标准是保护公共健康和环境安全的基础,对地下水的治理和管理起到了重要的指导作用。
4. 监测数据的分析与评估:监测数据由EPA和USGS等机构进行统一收集,并通过专业的地下水水质模型进行分析与评估。
这些模型能够模拟和预测地下水的流动和污染扩散趋势,提供决策者制定政策和规划资源的依据。
美国还与各州政府、学术机构和行业协会等建立了紧密的合作关系,共同推动地下水水质监测工作的开展。
各州政府负责本地区地下水的管理和监管工作,依据EPA的标准和指南制定相应的地方性法规,以适应本地区的实际情况。
美国对地下水的水质监测是一个涉及多个机构和部门的复杂工程,通过监测网络的建立、污染物的监测、质量标准的制定和数据分析与评估等手段,确保地下水的水质安全,保护公众健康和环境安全。
地下水污染脆弱性评价(一)
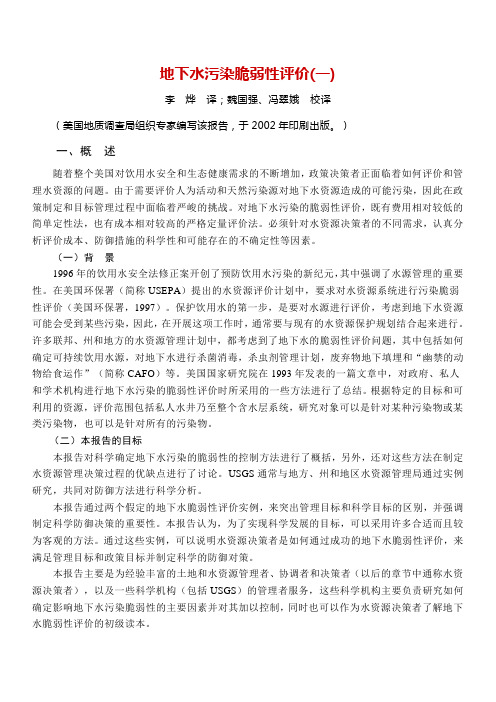
地下水污染脆弱性评价(一)李烨译;魏国强、冯翠娥校译(美国地质调查局组织专家编写该报告,于2002年印刷出版。
)一、概述随着整个美国对饮用水安全和生态健康需求的不断增加,政策决策者正面临着如何评价和管理水资源的问题。
由于需要评价人为活动和天然污染源对地下水资源造成的可能污染,因此在政策制定和目标管理过程中面临着严峻的挑战。
对地下水污染的脆弱性评价,既有费用相对较低的简单定性法,也有成本相对较高的严格定量评价法。
必须针对水资源决策者的不同需求,认真分析评价成本、防御措施的科学性和可能存在的不确定性等因素。
(一)背景1996年的饮用水安全法修正案开创了预防饮用水污染的新纪元,其中强调了水源管理的重要性。
在美国环保署(简称USEPA)提出的水资源评价计划中,要求对水资源系统进行污染脆弱性评价(美国环保署,1997)。
保护饮用水的第一步,是要对水源进行评价,考虑到地下水资源可能会受到某些污染,因此,在开展这项工作时,通常要与现有的水资源保护规划结合起来进行。
许多联邦、州和地方的水资源管理计划中,都考虑到了地下水的脆弱性评价问题,其中包括如何确定可持续饮用水源,对地下水进行杀菌消毒,杀虫剂管理计划,废弃物地下填埋和“幽禁的动物给食运作”(简称CAFO)等。
美国国家研究院在1993年发表的一篇文章中,对政府、私人和学术机构进行地下水污染的脆弱性评价时所采用的一些方法进行了总结。
根据特定的目标和可利用的资源,评价范围包括私人水井乃至整个含水层系统,研究对象可以是针对某种污染物或某类污染物,也可以是针对所有的污染物。
(二)本报告的目标本报告对科学确定地下水污染的脆弱性的控制方法进行了概括,另外,还对这些方法在制定水资源管理决策过程的优缺点进行了讨论。
USGS通常与地方、州和地区水资源管理局通过实例研究,共同对防御方法进行科学分析。
本报告通过两个假定的地下水脆弱性评价实例,来突出管理目标和科学目标的区别,并强调制定科学防御决策的重要性。
美国的地下水水质监测
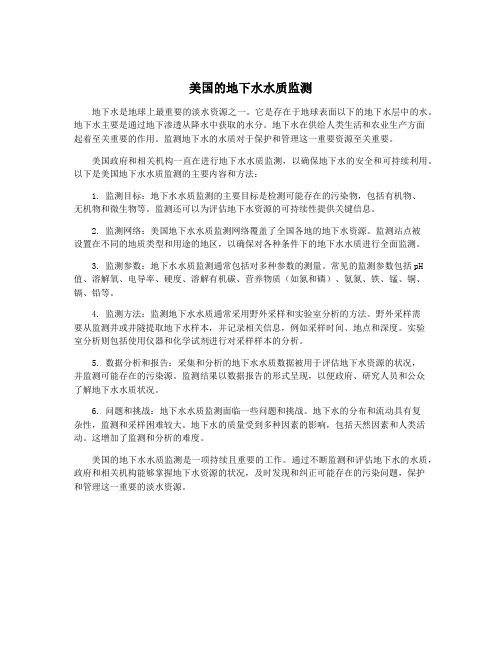
美国的地下水水质监测地下水是地球上最重要的淡水资源之一。
它是存在于地球表面以下的地下水层中的水。
地下水主要是通过地下渗透从降水中获取的水分。
地下水在供给人类生活和农业生产方面起着至关重要的作用。
监测地下水的水质对于保护和管理这一重要资源至关重要。
美国政府和相关机构一直在进行地下水水质监测,以确保地下水的安全和可持续利用。
以下是美国地下水水质监测的主要内容和方法:1. 监测目标:地下水水质监测的主要目标是检测可能存在的污染物,包括有机物、无机物和微生物等。
监测还可以为评估地下水资源的可持续性提供关键信息。
2. 监测网络:美国地下水水质监测网络覆盖了全国各地的地下水资源。
监测站点被设置在不同的地质类型和用途的地区,以确保对各种条件下的地下水水质进行全面监测。
3. 监测参数:地下水水质监测通常包括对多种参数的测量。
常见的监测参数包括pH 值、溶解氧、电导率、硬度、溶解有机碳、营养物质(如氮和磷)、氨氮、铁、锰、铜、镉、铅等。
4. 监测方法:监测地下水水质通常采用野外采样和实验室分析的方法。
野外采样需要从监测井或井隧提取地下水样本,并记录相关信息,例如采样时间、地点和深度。
实验室分析则包括使用仪器和化学试剂进行对采样样本的分析。
5. 数据分析和报告:采集和分析的地下水水质数据被用于评估地下水资源的状况,并监测可能存在的污染源。
监测结果以数据报告的形式呈现,以便政府、研究人员和公众了解地下水水质状况。
6. 问题和挑战:地下水水质监测面临一些问题和挑战。
地下水的分布和流动具有复杂性,监测和采样困难较大。
地下水的质量受到多种因素的影响,包括天然因素和人类活动。
这增加了监测和分析的难度。
美国的地下水水质监测是一项持续且重要的工作。
通过不断监测和评估地下水的水质,政府和相关机构能够掌握地下水资源的状况,及时发现和纠正可能存在的污染问题,保护和管理这一重要的淡水资源。
SWAT水文模型

SWAT水文模型介绍1概述SWAT(Soil and Water Assessment Tool)模型是美国农业部(USDA)农业研究局(ARS)开发的基于流域尺度的一个长时段的分布式流域水文模型。
它主要基于SWRRB模型,并吸取了CREAMS、GLEAMS、EPIC和ROTO的主要特征。
SWAT具有很强的物理基础,能够利用GIS和RS提供的空间数据信息模拟地表水和地下水的水量和水质,用来协助水资源管理,即预测和评估流域内水、泥沙和农业化学品管理所产生的影响。
该模型主要用于长期预测,对单一洪水事件的演算能力不强,模型主要由8个部分组成:水文、气象、泥沙、土壤温度、作物生长、营养物、农业管理和杀虫剂。
SWAT模型拥有参数自动率定模块,其采用的是等在1992年提出的SCE-UA算法。
模型采用模块化编程,由各水文计算模块实现各水文过程模拟功能,其源代码公开,方便用户对模型的改进和维护。
2模型原理SWAT模型在进行模拟时,首先根据DEM把流域划分为一定数目的子流域,子流域划分的大小可以根据定义形成河流所需要的最小集水区面积来调整,还可以通过增减子流域出口数量进行进一步调整。
然后在每一个子流域内再划分为水文响应单元HRU。
HRU 是同一个子流域内有着相同土地利用类型和土壤类型的区域。
每一个水文响应单元内的水平衡是基于降水、地表径流、蒸散发、壤中流、渗透、地下水回流和河道运移损失来计算的。
地表径流估算一般采用SCS径流曲线法。
渗透模块采用存储演算方法,并结合裂隙流模型来预测通过每一个土壤层的流量,一旦水渗透到根区底层以下则成为地下水或产生回流。
在土壤剖面中壤中流的计算与渗透同时进行。
每一层土壤中的壤中流采用动力蓄水水库来模拟。
河道中流量演算采用变动存储系数法或马斯金根演算法。
模型中提供了三种估算潜在蒸散发量的计算方法—Hargreaves、Priestley-Taylor和Penman-Monteith。
每一个子流域内侵蚀和泥沙量的估算采用改进的USLE方程,河道内泥沙演算采用改进的Bagnold泥沙运移方程。
美国农药生态风险评价技术
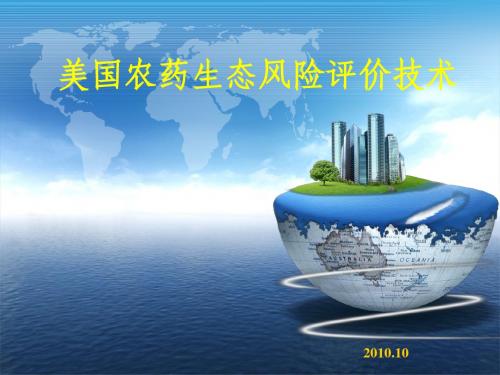
水生生物风险商值的计算方法
USEPA农 药生态 风险评 价的常 用模型
4.风险表征
风险表征(Risk Characterization)是对 暴露于各种胁迫之下的不利生态效应的综 合判断和表达,是生态风险评价的最后一个 阶段。目前, USEPA大多采用商值法进行风 险表征。商值法又称比率法,是使用最普遍、 最广泛的风险表征方法。
USEPA所采用的商值法其基本原理是把实际 监测或由假设估算、模型预测得出的污染物环境 浓度(环境暴露浓度, EEC)与实验室测得的表征该 物质危害的毒性数据(如LC50)相比,得到风险商值 (Risk Quotient, RQ) [水生生物风险商值的计算方 法见式(1)、式(2)]。将风险商值与OPP制定的风 险关注标准(Levels of Concern, LOCs)进行比较, 从而对农药的生态风险作出初步的判断。LOCs是 确定风险等级的判别标准,不同的风险商值分别对 应不同的关注标准,不同的关注标准又分别对应于 不同的风险等级,各风险等级又对应着不同的管理 措施。如果风险商值超过了相应的关注标准,则表 示具有相应的风险,就需要采取相应的管理措施。 表2是USEPA农药项目办公室制定的水生动物关 注标准。
美 国 国 家 环 境 保 护 局 (U.S. Environmental Protection Agency, USEPA )于1998年制定的《生态风险 评价准则》是世界上最早的生态风险 评价方面的指导文件。该准则将生态 风险评价过程分为3个主要阶段:问题 表述、分析和风险表征。美国的农药 / 生态风险评价也遵循这一程序。同时 又根据不同的保护目标,建立了相应 的风险评价技术,包括农药对地表水 水生生物、对陆生生物以及地下水的 风险评价技术。
水生生态风险评价是生态风险评价的 重要组成部分,应用水生生态风险评价技术 可以预测或评估农药使用可能对水生生态 环境及水生生物产生的危害和影响。一般 而言,在生态风险评价中,较为关键的过程 主要包括:生态受体的选择、评价终点的确 定、暴露评价方法及风险表征方法的选择。
SWAT模型在农业面源污染研究中的应用
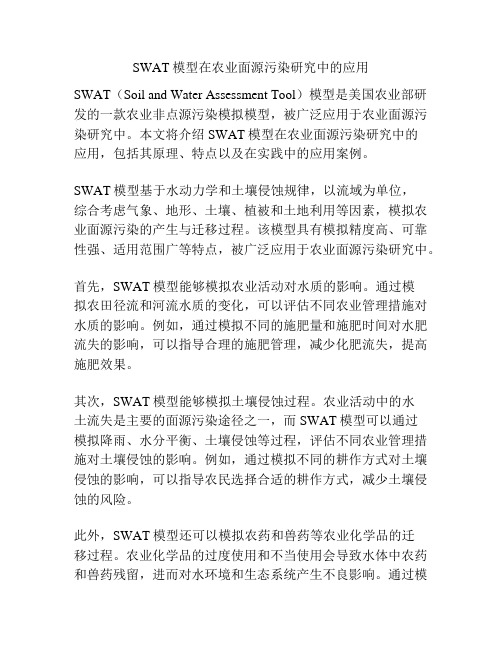
SWAT模型在农业面源污染研究中的应用SWAT(Soil and Water Assessment Tool)模型是美国农业部研发的一款农业非点源污染模拟模型,被广泛应用于农业面源污染研究中。
本文将介绍SWAT模型在农业面源污染研究中的应用,包括其原理、特点以及在实践中的应用案例。
SWAT模型基于水动力学和土壤侵蚀规律,以流域为单位,综合考虑气象、地形、土壤、植被和土地利用等因素,模拟农业面源污染的产生与迁移过程。
该模型具有模拟精度高、可靠性强、适用范围广等特点,被广泛应用于农业面源污染研究中。
首先,SWAT模型能够模拟农业活动对水质的影响。
通过模拟农田径流和河流水质的变化,可以评估不同农业管理措施对水质的影响。
例如,通过模拟不同的施肥量和施肥时间对水肥流失的影响,可以指导合理的施肥管理,减少化肥流失,提高施肥效果。
其次,SWAT模型能够模拟土壤侵蚀过程。
农业活动中的水土流失是主要的面源污染途径之一,而SWAT模型可以通过模拟降雨、水分平衡、土壤侵蚀等过程,评估不同农业管理措施对土壤侵蚀的影响。
例如,通过模拟不同的耕作方式对土壤侵蚀的影响,可以指导农民选择合适的耕作方式,减少土壤侵蚀的风险。
此外,SWAT模型还可以模拟农药和兽药等农业化学品的迁移过程。
农业化学品的过度使用和不当使用会导致水体中农药和兽药残留,进而对水环境和生态系统产生不良影响。
通过模拟农业化学品的迁移过程,可以评估农业活动对水体中农药和兽药的污染风险,并探讨相应的农业管理措施。
例如,通过模拟不同农业管理措施对农药和兽药的损失和迁移的影响,可以优化农业管理策略,减少农药和兽药对水体的污染。
在实践中,SWAT模型已经在许多国内外研究中得到了广泛应用。
以中国为例,研究者利用SWAT模型研究了不同耕作方式、不同施肥管理措施、不同农田排水管理方式等对农业面源污染的影响。
通过模拟和分析,得出了一系列合理的管理建议,如调整施肥时间、减少施肥量、改善排水系统等,以减少农业面源污染对水环境的影响。
地下水污染风险评价的综合模煳随机模拟方法
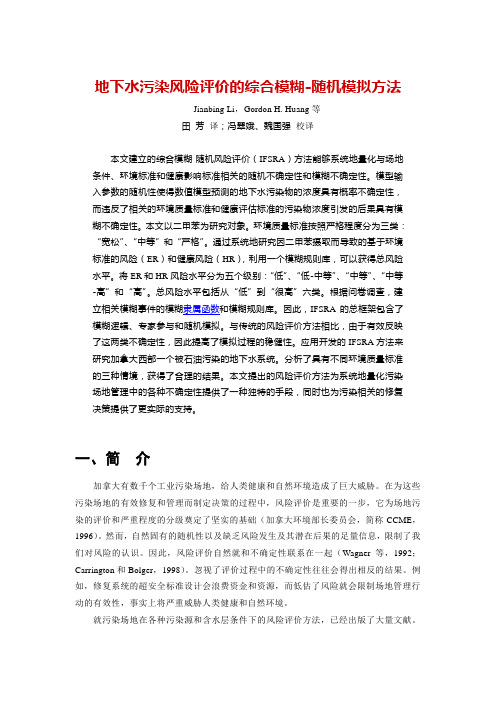
地下水污染风险评价的综合模糊-随机模拟方法Jianbing Li,Gordon H. Huang等田芳译;冯翠娥、魏国强校译本文建立的综合模糊-随机风险评价(IFSRA)方法能够系统地量化与场地条件、环境标准和健康影响标准相关的随机不确定性和模糊不确定性。
模型输入参数的随机性使得数值模型预测的地下水污染物的浓度具有概率不确定性,而违反了相关的环境质量标准和健康评估标准的污染物浓度引发的后果具有模糊不确定性。
本文以二甲苯为研究对象。
环境质量标准按照严格程度分为三类:“宽松”、“中等”和“严格”。
通过系统地研究因二甲苯摄取而导致的基于环境标准的风险(ER)和健康风险(HR),利用一个模糊规则库,可以获得总风险水平。
将ER和HR风险水平分为五个级别:“低”、“低-中等”、“中等”、“中等-高”和“高”。
总风险水平包括从“低”到“很高”六类。
根据问卷调查,建立相关模糊事件的模糊隶属函数和模糊规则库。
因此,IFSRA的总框架包含了模糊逻辑、专家参与和随机模拟。
与传统的风险评价方法相比,由于有效反映了这两类不确定性,因此提高了模拟过程的稳健性。
应用开发的IFSRA方法来研究加拿大西部一个被石油污染的地下水系统。
分析了具有不同环境质量标准的三种情境,获得了合理的结果。
本文提出的风险评价方法为系统地量化污染场地管理中的各种不确定性提供了一种独特的手段,同时也为污染相关的修复决策提供了更实际的支持。
一、简介加拿大有数千个工业污染场地,给人类健康和自然环境造成了巨大威胁。
在为这些污染场地的有效修复和管理而制定决策的过程中,风险评价是重要的一步,它为场地污染的评价和严重程度的分级奠定了坚实的基础(加拿大环境部长委员会,简称CCME,1996)。
然而,自然固有的随机性以及缺乏风险发生及其潜在后果的足量信息,限制了我们对风险的认识。
因此,风险评价自然就和不确定性联系在一起(Wagner等,1992;Carrington和Bolger,1998)。
epa评估方法
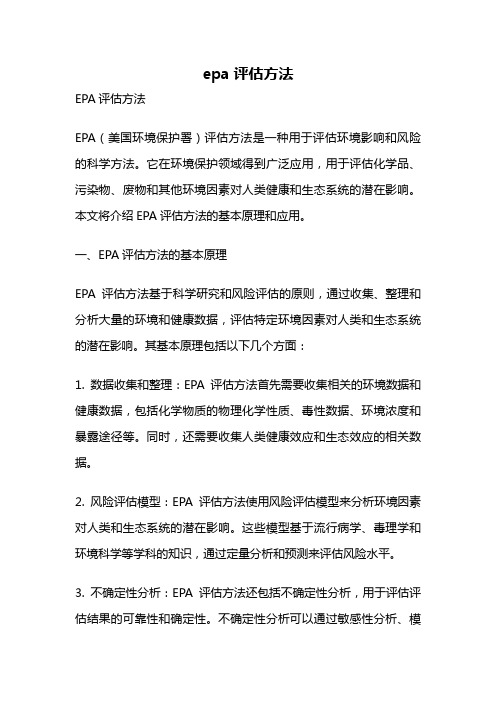
epa评估方法EPA评估方法EPA(美国环境保护署)评估方法是一种用于评估环境影响和风险的科学方法。
它在环境保护领域得到广泛应用,用于评估化学品、污染物、废物和其他环境因素对人类健康和生态系统的潜在影响。
本文将介绍EPA评估方法的基本原理和应用。
一、EPA评估方法的基本原理EPA评估方法基于科学研究和风险评估的原则,通过收集、整理和分析大量的环境和健康数据,评估特定环境因素对人类和生态系统的潜在影响。
其基本原理包括以下几个方面:1. 数据收集和整理:EPA评估方法首先需要收集相关的环境数据和健康数据,包括化学物质的物理化学性质、毒性数据、环境浓度和暴露途径等。
同时,还需要收集人类健康效应和生态效应的相关数据。
2. 风险评估模型:EPA评估方法使用风险评估模型来分析环境因素对人类和生态系统的潜在影响。
这些模型基于流行病学、毒理学和环境科学等学科的知识,通过定量分析和预测来评估风险水平。
3. 不确定性分析:EPA评估方法还包括不确定性分析,用于评估评估结果的可靠性和确定性。
不确定性分析可以通过敏感性分析、模拟和统计方法等来进行。
二、EPA评估方法的应用EPA评估方法广泛应用于环境监测、风险评估和决策支持等领域。
其主要应用包括以下几个方面:1. 环境污染评估:EPA评估方法可以用于评估污染物对环境的潜在影响。
通过收集环境数据和污染物的毒性数据,结合风险评估模型,可以评估污染物的暴露水平和潜在风险。
2. 化学品评估:EPA评估方法可以用于评估化学品对人类健康和环境的潜在影响。
通过收集化学品的物理化学性质、毒性数据和暴露途径等信息,结合风险评估模型,可以评估化学品的致癌、致畸和致突变等潜在风险。
3. 废物管理评估:EPA评估方法可以用于评估废物对环境和人类健康的潜在影响。
通过收集废物的组成、毒性数据和处理方式等信息,结合风险评估模型,可以评估废物的处理风险和环境影响。
4. 环境政策制定:EPA评估方法可以用于支持环境政策的制定和实施。
地下水污染风险的主要评估方法
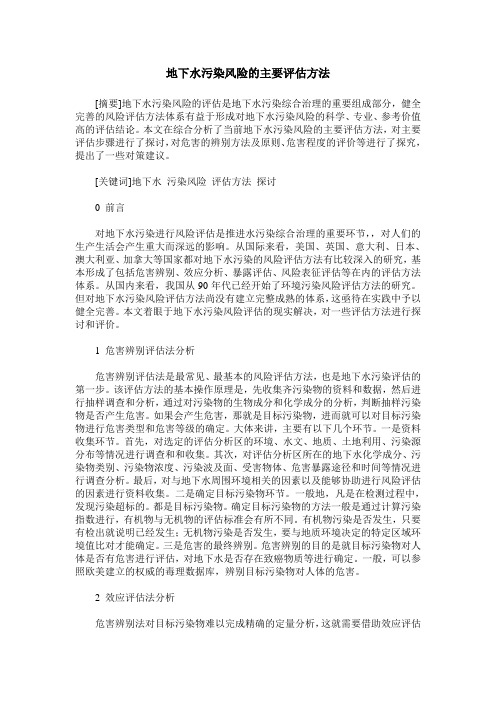
地下水污染风险的主要评估方法[摘要]地下水污染风险的评估是地下水污染综合治理的重要组成部分,健全完善的风险评估方法体系有益于形成对地下水污染风险的科学、专业、参考价值高的评估结论。
本文在综合分析了当前地下水污染风险的主要评估方法,对主要评估步骤进行了探讨,对危害的辨别方法及原则、危害程度的评价等进行了探究,提出了一些对策建议。
[关键词]地下水污染风险评估方法探讨0 前言对地下水污染进行风险评估是推进水污染综合治理的重要环节,,对人们的生产生活会产生重大而深远的影响。
从国际来看,美国、英国、意大利、日本、澳大利亚、加拿大等国家都对地下水污染的风险评估方法有比较深入的研究,基本形成了包括危害辨别、效应分析、暴露评估、风险表征评估等在内的评估方法体系。
从国内来看,我国从90年代已经开始了环境污染风险评估方法的研究。
但对地下水污染风险评估方法尚没有建立完整成熟的体系,这亟待在实践中予以健全完善。
本文着眼于地下水污染风险评估的现实解决,对一些评估方法进行探讨和评价。
1 危害辨别评估法分析危害辨别评估法是最常见、最基本的风险评估方法,也是地下水污染评估的第一步。
该评估方法的基本操作原理是,先收集齐污染物的资料和数据,然后进行抽样调查和分析,通过对污染物的生物成分和化学成分的分析,判断抽样污染物是否产生危害。
如果会产生危害,那就是目标污染物,进而就可以对目标污染物进行危害类型和危害等级的确定。
大体来讲,主要有以下几个环节。
一是资料收集环节。
首先,对选定的评估分析区的环境、水文、地质、土地利用、污染源分布等情况进行调查和和收集。
其次,对评估分析区所在的地下水化学成分、污染物类别、污染物浓度、污染波及面、受害物体、危害暴露途径和时间等情况进行调查分析。
最后,对与地下水周围环境相关的因素以及能够协助进行风险评估的因素进行资料收集。
二是确定目标污染物环节。
一般地,凡是在检测过程中,发现污染超标的。
都是目标污染物。
确定目标污染物的方法一般是通过计算污染指数进行,有机物与无机物的评估标准会有所不同。
运用SI-CGROW模型预测农药对地下水的污染风险
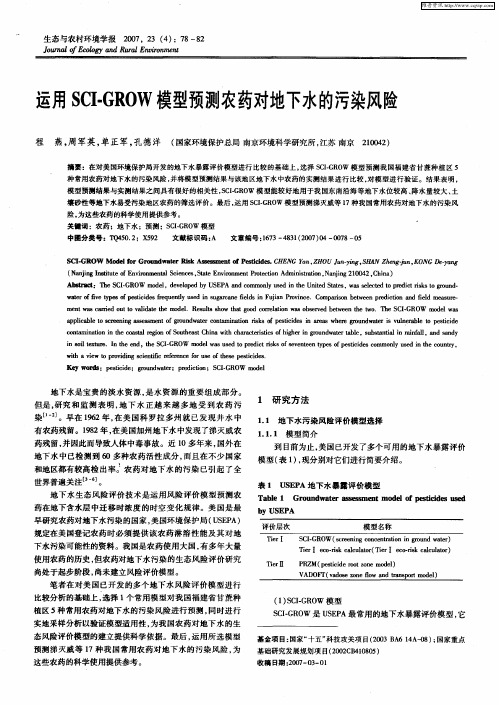
地下水是宝贵 的淡水资 源 , 水资 源的重要 组成 部分。 是 但是 , 究和 监 测表 明, 下 水 正越 来越 多 地 受 到农 药 污 研 地
程 燕 , 周军英 , 单正军, 孔德洋 ( 环境保护总 南京环境科学研究所, 南京 2 02 国家 局 江苏 1 4) 0
摘要:在对美 国环境保 护局开发 的地下水暴露评价模型进行 比较 的基础上 , 择 S I R W 模型预测我 国福建省 甘蔗种植 区 5 选 C. O G
种 常用农药对地下水的污染风险 , 并将模 型预测结果与该地区地下水中农药的实测结果进行 比较 , 对模 型进行验证。结果表 明,
模 型预测结果与实测结果之间具有很好的相关性 ,C— R W 模型能较好地用于我 国东南沿海等地下水位较高 、 SI O G 降水量较大 、 土 壤砂性等地下水易受污染地区农药的筛选评价 。最后 , 运用 S I R W 模型预测涕灭威等 l C. O G 7种我 国常用农药对地下水 的污染风 险, 为这些农药的科学使用提供参考。
关键词 :农药 ;地下水 ;预测 ;S I O C. W模 型 GR 中圈分类号 :T 40 2 (9 Q 5 . ;) 2 5 文献标 识码 : A 文章编号 :6 3— 8 1 2 0 ) 4—07 0 17 4 3 ( 07 0 0 8— 5
S - OW M oe frGru d t CIGR d lo o n wae s ses n fP sid s C E G Y n Z OUJ nyn S A g egjn K NGD -ag rRi kA ssmeto et e. H N a , H u—lg,H N hn - , O d u ey n
me t sc rid o tt aiaetemo 1 i wa r are u ov l t h de.Reut h w a o d c reain w so sre ewe nte to T eS ・ d sl s o t tgo orlto a be vd b t e . h CIGROW d e s s h h w mo lwa a pia l os re igassme to ru d trc na n t n r k fp s cd si ra eego n wae sv l rbet siie p l bet ce nn ses n fgo n wae o tmia o s so et ie n ae swh r ru d t ri unea l op tcd c i i i e
hspf模型
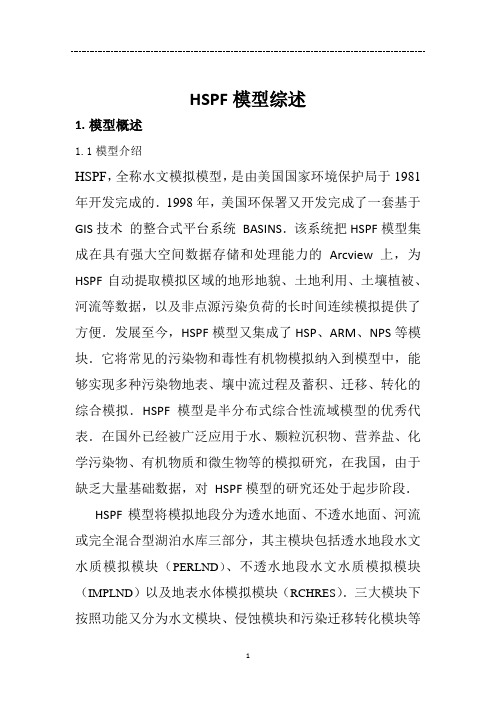
HSPF模型综述1.模型概述1.1模型介绍HSPF,全称水文模拟模型,是由美国国家环境保护局于1981年开发完成的.1998年,美国环保署又开发完成了一套基于GIS技术的整合式平台系统BASINS.该系统把HSPF模型集成在具有强大空间数据存储和处理能力的Arcview上,为HSPF自动提取模拟区域的地形地貌、土地利用、土壤植被、河流等数据,以及非点源污染负荷的长时间连续模拟提供了方便.发展至今,HSPF模型又集成了HSP、ARM、NPS等模块.它将常见的污染物和毒性有机物模拟纳入到模型中,能够实现多种污染物地表、壤中流过程及蓄积、迁移、转化的综合模拟.HSPF模型是半分布式综合性流域模型的优秀代表.在国外已经被广泛应用于水、颗粒沉积物、营养盐、化学污染物、有机物质和微生物等的模拟研究,在我国,由于缺乏大量基础数据,对HSPF模型的研究还处于起步阶段.HSPF模型将模拟地段分为透水地面、不透水地面、河流或完全混合型湖泊水库三部分,其主模块包括透水地段水文水质模拟模块(PERLND)、不透水地段水文水质模拟模块(IMPLND)以及地表水体模拟模块(RCHRES).三大模块下按照功能又分为水文模块、侵蚀模块和污染迁移转化模块等子模块,可以实现对径流、颗粒沉积物、营养盐、化学污染物、有机物质和微生物等的连续模拟(见图1).图1 HSPF模型结构与功能1.2模型基础数据库模型需要的输入数据主要有:(1)流域的数字高程模型(DEM).用来划分子流域和确定出流路径;(2)土地利用数据.主要用来计算植被生长、耗水和地表产汇流;(3)土壤数据.用来计算壤中流和浅层地下水量;(4)气象数据,包括日降雨资料、日最高最低气温、风速、日辐射量、相对湿度、气温站位置高程、雨量站位置高程等,用来计算流量和蒸散发量n1;(5)农业管理措施和水库和湖泊位置、出流点等;(6)监测站数据.包括各水质参数数据,例如总氮、总磷、BOD等;(7)社会经济数据,包括人口普查数据、人口密度数据等.1.3水文过程模拟HSPF模型水文模块在非点源模型中是最为完善的,它以StanfordIV机理模型为基础,将研究区域分为透水地面和不透水地面两种类型,针对不同地面水文过程进行模拟.模型将研究区域自上而下分为树冠层、植被层和各土壤层(包括表层土壤、上土壤层、下土壤层和地下水涵养层).降水在这些垂直的存储层间进行分配.透水地面的模拟考虑降雨或降雪、截留、地表填洼、渗透、蒸散发、地表径流、壤中流和地下水流等水文过程.降雨或降雪被地面截留一部分,再扣除地表填洼、下渗、蒸发,最后形成地表径流.不透水地面的模拟考虑降雨或降雪、截留、蒸散发、地表径流.降雨或降雪经扣除屋顶集水、沥青变湿及植被截留后形成地表径流.降雨最终由地表径流、壤中流和地下水流进入河流.1.4泥沙侵蚀模拟相比目前很多模型釆用的通用土壤流失方程(USLE),HSPF 模型对泥沙侵蚀的模拟更具有机理性.它将侵蚀过程分为雨滴溅蚀、径流冲刷和径流运移等若干子过程,分别对其进行模拟.泥沙侵蚀模拟过程包括降雨对透水地面土壤的剥蚀,对不透水地面的冲刷以及地表径流对泥沙的输移过程.用于模拟泥沙剥蚀和迁移过程的数学方程是基于Meyer和Wischmeier 所提出的降雨对土壤表面侵蚀的算法.泥沙随水流的演进输移,HSPF模型采用Toffaleti 、collby 或幂函数法以及临界切应力原理进行模拟.泥沙的传输按照泥沙粒径大小,粉沙和粘粒的传输、沉降和冲刷根据临界剪切应力原理判断产生沉积或是冲刷,沙粒的传输可以用Toffaleti、collby 或幂级数函数法来计算.1.5污染物迁移模型HSPF模型污染物迁移模块考虑了污染物在多种环境介质之间的迁移转化过程,考虑了污染物在土壤中的状态、含量,及其受到各种物理化学过程及生物过程的影响,可以模拟输出BOD、DO、营养物、农药和微生物等多种污染物负荷.尤其对氮的模拟,模型综合考虑了溶解态,吸附态氮,有机氮和无机氮,氮素间的相互转化,以及氮素与环境介质间的迁移等多个过程.1.6模型的适用性HSPF模型结合了分布式流域水文模型和其它非分布式流域模型的一些优点,是一个可以模拟流域内连续的水文过程以及水质变化过程的模型.①模型集成于BASINS系统平台,实现了模拟区域地形地貌、土地利用、土壤植被、河流等数据的自动提取.与SWAT模型相比,它包含融雪模块,因此对冬季径流的模拟具有优势.②对于降雨径流,HSPF模型能够将降水径流过程按某一尺度进行空间划分,对每一区域降雨、下渗等过程分别进行动态和连续的模拟.③对于子流域,HSPF模型每个子流域间具有承接关系,并可根据不同需要调整子流域水文响应单元大小.既实现了分布式模拟,又能减少计算冗余,同时避免了类似分布式的结构假定函数与实际不符而造成的错误.④对于模拟尺度,HSPF模型主要用于农业和城市混合型的不同时空尺度流域,能够模拟时间尺度为小时的产汇流过程.模型中WD-Mutil 软件可将现有气候气象数据进行衍生和扩充,延长了模拟时间序列.2 国内外HSPF模型的研究进展2.1水文过程与情景模拟分析许多研究表明,HSPF模型在不同地区的水文过程模拟中均有很好的表现.Alarcon等利用HSPF 模型模拟墨西哥湾北部Mobile Bay流域的水文过程,结果表现很好。
土壤污染健康风险评估模型
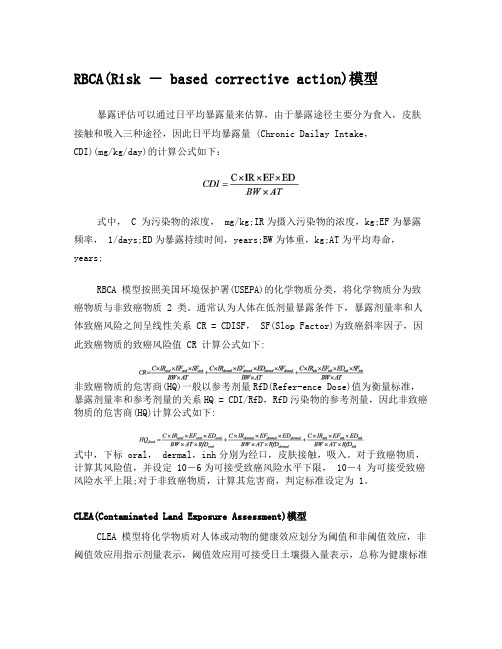
RBCA(Risk - based corrective action)模型暴露评估可以通过日平均暴露量来估算,由于暴露途径主要分为食入,皮肤接触和吸入三种途径,因此日平均暴露量 (Chronic Dailay Intake,CDI)(mg/kg/day)的计算公式如下:式中, C 为污染物的浓度, mg/kg;IR为摄入污染物的浓度,kg;EF为暴露频率, 1/days;ED为暴露持续时间,years;BW为体重,kg;AT为平均寿命,years;RBCA 模型按照美国环境保护署(USEPA)的化学物质分类,将化学物质分为致癌物质与非致癌物质 2 类。
通常认为人体在低剂量暴露条件下,暴露剂量率和人体致癌风险之间呈线性关系 CR = CDISF, SF(Slop Factor)为致癌斜率因子,因此致癌物质的致癌风险值 CR 计算公式如下:非致癌物质的危害商(HQ)一般以参考剂量RfD(Refer-ence Dose)值为衡量标准,暴露剂量率和参考剂量的关系HQ = CDI/RfD,RfD污染物的参考剂量,因此非致癌物质的危害商(HQ)计算公式如下:式中,下标 oral, dermal,inh分别为经口,皮肤接触,吸入。
对于致癌物质,计算其风险值,并设定 10-6为可接受致癌风险水平下限, 10-4 为可接受致癌风险水平上限;对于非致癌物质,计算其危害商,判定标准设定为 1。
CLEA(Contaminated Land Exposure Assessment)模型CLEA 模型将化学物质对人体或动物的健康效应划分为阈值和非阈值效应,非阈值效应用指示剂量表示,阈值效应用可接受日土壤摄入量表示,总称为健康标准值(HCV).依据日平均暴露量(CDI)与 HCV 的比值来评价化学物质的危害程度。
ADE/HCV 的计算公式如下:式中, C 为污染物的浓度, mg/kg;IR为摄入污染物的浓度,kg;EF为暴露频率,1/days;ED为暴露持续时间,years;BW为体重,kg;AT为平均寿命, years; HCV 为健康标准值,单位为 mg/kg/d;下标 oral, dermal,inh分别为经口,皮肤接触,吸入。
计算土壤和地下水风险控制值的推荐模型
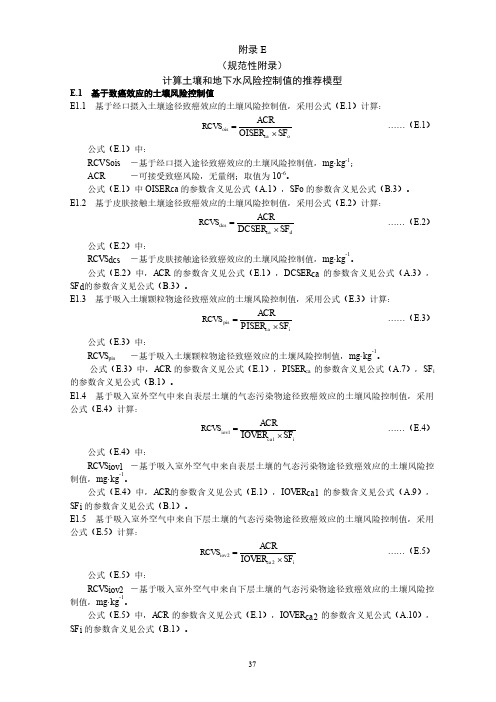
RCVGcgw
ACR CGWERca
SFo
……(E.18)
公式(E.18)中: RCVGcgw -基于饮用地下水途径致癌效应的地下水风险控制值,mg·L-1。 公式(E.18)中,ACR 的参数含义见公式(E.1),CGWERca 的参数含义见公式(A.19), SFo 的参数含义见公式(B.3)。 E4.4 基于 3 种地下水暴露途径综合致癌效应的地下水风险控制值,采用公式(E.19)计算:
E2.1 基于经口摄入土壤途径非致癌效应的土壤风险控制值,采用公式(E.8)计算:
HCVSois
RfDo
SAF OISERnc
AHQ
……(E.8)
公式(E.8)中: HCVSois -基于经口摄入土壤途径非致癌效应的土壤风险控制值,mg·kg-1;
AHQ
-可接受危害商,无量纲;取值为 1。
公式(E.8)中,RfDo 的参数含义见公式(B.4),OISERnc 的参数含义见公式(A.2),
HCVS pis
RfDi
SAF AHQ PISERnc
……(E.10)
公式(E.10)中: HCVSpis -基于吸入土壤颗粒物途径非致癌效应的土壤风险控制值,mg·kg-1。 公式(E.10)中,RfDi 的参数含义见公式(B.2),AHQ 的参数含义见公式(E.8), PISERnc的参数含义见公式(A.8),SAF 的参数含义见公式(C.8)。
HCVSn
OISERnc
DCSERnc
AHQ SAF PISERnc IOVERnc1
IOVERnc 2
IIVERnc1
RfDo
RfDd
RfDi
……(E.14)
公式(E.14)中:
- 1、下载文档前请自行甄别文档内容的完整性,平台不提供额外的编辑、内容补充、找答案等附加服务。
- 2、"仅部分预览"的文档,不可在线预览部分如存在完整性等问题,可反馈申请退款(可完整预览的文档不适用该条件!)。
- 3、如文档侵犯您的权益,请联系客服反馈,我们会尽快为您处理(人工客服工作时间:9:00-18:30)。
美国环保署农药地下水风险评估模型文伯健,李文娟*,程敏(中国农业科学院农业资源与农业区划研究所,北京100081)收稿日期:2013-07-16基金项目:公益性行业(农业)科研专项“农药风险评估综合配套技术研究”(20090354-05)作者简介:文伯健(1986—),男,四川安岳人,硕士研究生,主要从事农药地下水风险评估研究。
E-mail :wenbojian@ *通信作者:李文娟E-mail :liwenjuan@ 摘要:在介绍美国农药环境风险评估的概念、分级、地下水农药监测情况及水资源的立法保护等基础上,重点阐述了美国环保署在农药登记管理过程中使用的2个地下水风险评估模型,即SCI-GROW 和PRZM-GW 模型。
SCI-GROW 是以好氧条件土壤半衰期和土壤有机碳分配系数为自变量的经验线性回归模型,而PRZM-GW 则是描述农药在土壤中运动的一维、有限差分模型。
本文通过对美国环保署这2个特点鲜明的模型的介绍,希望能为我国的农药地下水风险评估及模型的开发提供一个新视角。
关键词:农药;环境风险评估;模型;SCI-GROW ;PRZM-GW ;美国中图分类号:X820.4文献标志码:A 文章编号:2095-6819(2013)06-0068-06The Groundwater Risk Assessment Models for Pesticide for USEPAWEN Bo-jian,LI Wen-juan *,CHENG Min(Institute of Agricultural Resources and Regional Planning,Chinese Academy of Agricultural Sciences,Beijing 100081,China )Abstract :Groundwater risk assessment of pesticide in the USA is characteristic.A profile of the groundwater risk assessment of pesticide in the USA was introduced,including the concepts and the tiers of the groundwater risk assessment,the results of groundwater monitoring,the legislations of water resource protection,and etc.The groundwater risk assessment models,SCI-GROW and PRZM-GW,which are used in pesticide management by USEPA were elaborated.SCI-GROW is an empirical linear regression model that takes aerobic soil metabolism half-life and soil organic carbon partition coefficient as the independent variables.PRZM-GW is a one-dimensional,finite-difference model.The paper aims to provide new vision in groundwater risk assessment of pesticide for developing our own models that fit China ′s agricultural practice and pesticide application.Keywords :pesticide;environmental risk assessment;model;SCI-GROW;PRZM-GW ;USA农业资源与环境学报2013年12月·第30卷·第6期:68-73December 2013·Vol.30·No.6:68-73Journal of Agricultural Resources and Environment如何有效地控制农药对地下水构成的风险是一个引起广泛关注的问题。
农业生产过程中,总有一定比例的农药会在施用后通过淋溶进入地下水。
地下水是世界上很多地区的饮用水源,因此当农药在地下水中的浓度超过一定限量时,就会对人类健康造成风险。
由于地下水的地理特征,地下水的污染治理十分困难。
利用暴露模型对农药地下水风险进行快速有效的评估,根据评估结果进行严格的农药登记,是防患于未然的有效途径之一。
世界上很多发达国家和地区都已建立各自的农药环境风险评估体系,开发出各具特色的风险评估模型。
其中,以欧盟和美国的模型最具代表性。
欧盟的模型开发者大多遵守反吝啬原则(Anti-parsimony Prin -ciple ),认为模型的内涵应当足够丰富以正确反映事物联系的复杂性。
因此,在欧盟的模型构建过程中,选取的变量全面,开发出的模型也比较复杂,比如欧盟的农药地下水风险评估模型PEARL (Pesticide Emis -sion Assessment at Regional and Local scales )。
美国模型开发者一般秉承吝啬原则(Law of Parsimony ),认为如果某一因素足以解释事物的特性,则不应当接受比这更多的因素。
因此,在美国的模型构建过程中,选取的变量较少,开发出的模型相对精简,例如美国的农2013年12月药地下水风险评估模型SCI-GROW(Screening Con-centration in Ground Water)和PRZM-GW(Pesticide Root Zone Model-Ground Water)。
本文将对美国环保署(U.S.Environmental Protection Agency,USEPA)农药地下水风险评估的相关内容及使用的模型SCI-GROW和PRZM-GW进行介绍。
1美国农药地下水风险评估相关概念USEPA把农药环境风险评估(Pesticide Environ-mental Risk Assessment)定义为就农药对生态环境产生不良效应的可能性进行评估的过程,该过程分为问题描述(Problem Formulation)、分析(Analysis)和风险表征(Risk Characterization)3个部分。
问题描述需要确定保护目标,收集必要的数据信息,制定风险分析和表征计划,得到评估终点和概念模型;分析是指通过将数据进行评估,确定暴露可能的发生方式和程度(暴露分析)和预期发生的生态效应的类型和程度(生态效应分析);风险表征是对各种假设、不确定性、分析的有效性和局限性进行总结,最终得到关于生态危害、不确定性和支持证据的风险描述[1]。
在进行农药水环境风险评估时,国际上多采用分级的评估方法,即由初级风险评估向高级风险评估过渡。
如果初级风险评估的结果显示某种农药没有不可接受的风险,即终止评估,予以登记;如果初级风险评估结果显示某种农药具有不可接受的风险,则需要进入更高一级的风险评估。
初级风险评估比较保守,预测浓度往往比实际环境中的浓度要高很多。
高级风险评估需要更多的数据、使用更复杂的模型或进行实际监控研究,其评估结果更接近农药的实际应用情况[2]。
美国农药水环境风险评估分为4级(Tier),每级的结构相近,都包括问题描述、分析和风险表征3个部分。
TierⅠ:筛选。
初级评估主要需筛选出低风险农药,通过保守的模型预测来识别农药对水环境(以敏感物种为代表)可能构成的风险。
TierⅡ:包括基本时空因子的风险表征。
在TierⅠ的基础上提供更详细的农药的物理、化学和环境行为数据,评估农药使用中由于环境条件变化而造成的风险随时间、空间而变化的情况。
TierⅢ:精确估计风险及其不确定性。
在TierⅡ的基础上进行更细致、精确的工作,包括慢性毒性研究、实验室或田间模拟环境行为研究、制定详尽的风险管理措施。
TierⅣ:复杂的模拟和减少风险措施的有效性研究,常包括多方面的试验和监测计划,以确切地描述毒性或暴露的关键方面的特征[3]。
2美国地下水农药监测及相关立法20世纪70年代美国便在其地下水中发现了农药残留,并随后开始对地下水中的农药进行系统地调查与监测。
1979年,美国在加利福尼亚州的井水中发现二溴氯丙烷,在纽约的井水中发现灭敌威。
1986年,美国在其24个州的地下水监测中,共计检出19种农药,并因此关闭了数千口饮用水井[4]。
1992—1999年,美国地质调查局(U.S.Geological Survey,USGS)对全美1255口家庭水井和242口公共水井的水质调查结果表明,农药的检出率为38%(检出标准为0.1μg·L-1),超过美国最大污染浓度(Maximum Contami-nant Level,MCL)的农药有4种,其中狄氏剂检出率最高,在公共水井中的检出率达1.3%[5]。
1993—2007年,USGS对全美932个公共水井的水质监测结果显示,检出率超过1%(检出标准为0.1μg·L-1)的农药及其代谢产物有4种,其中,莠去津的检出率达2.0%[6]。
农药在地下水中被相继检出,引起了美国公众的广泛关注,促使美国在立法层面控制农药对地下水构成的风险。
1988年,美国通过《联邦杀虫剂、杀菌剂、灭鼠剂法案》(The Federal Insecticide,Fungicide and Rodenticide Act,FIFRA)修正案,要求在农药进入市场前,农药厂家必须向USEPA提供农药的相关数据,以评估农药对地下水的淋溶风险[7]。
《饮用水安全法》(The Safe Drinking Water Act,SDWA)1996年修正案则明确要求保护包括地下水在内的饮用水源,要求USEPA逐步制定饮用水中污染物浓度(包括农药)的法定限量[8]。
1996年,美国通过《食品质量保护法》(The Food Quality Protection Act,FQPA),要求USEPA 对美国现正在使用的上百种农药对地下水(饮用水源)的风险进行快速、定量评估,该法案直接推动了农药地下水风险评估模型在USEPA农药登记管理过程中的应用[9]。
3USEPA农药地下水风险评估模型USEPA农药环境风险评估模型分为水环境模型、陆地环境模型、大气模型和健康影响模型4类。