COMSOL官方实例解析
comsol 案例
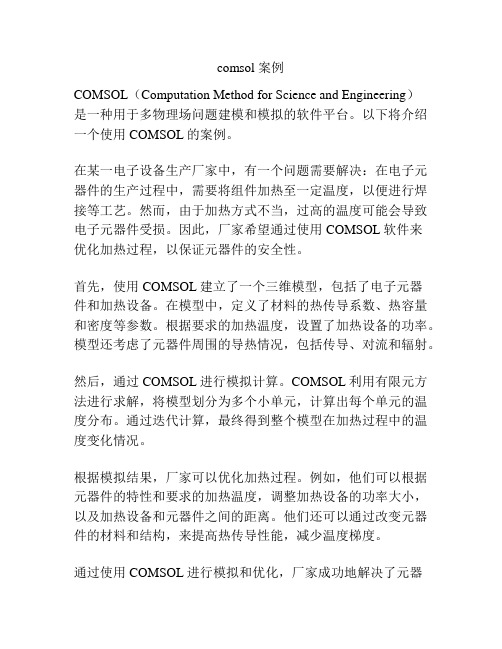
comsol 案例COMSOL(Computation Method for Science and Engineering)是一种用于多物理场问题建模和模拟的软件平台。
以下将介绍一个使用COMSOL的案例。
在某一电子设备生产厂家中,有一个问题需要解决:在电子元器件的生产过程中,需要将组件加热至一定温度,以便进行焊接等工艺。
然而,由于加热方式不当,过高的温度可能会导致电子元器件受损。
因此,厂家希望通过使用COMSOL软件来优化加热过程,以保证元器件的安全性。
首先,使用COMSOL建立了一个三维模型,包括了电子元器件和加热设备。
在模型中,定义了材料的热传导系数、热容量和密度等参数。
根据要求的加热温度,设置了加热设备的功率。
模型还考虑了元器件周围的导热情况,包括传导、对流和辐射。
然后,通过COMSOL进行模拟计算。
COMSOL利用有限元方法进行求解,将模型划分为多个小单元,计算出每个单元的温度分布。
通过迭代计算,最终得到整个模型在加热过程中的温度变化情况。
根据模拟结果,厂家可以优化加热过程。
例如,他们可以根据元器件的特性和要求的加热温度,调整加热设备的功率大小,以及加热设备和元器件之间的距离。
他们还可以通过改变元器件的材料和结构,来提高热传导性能,减少温度梯度。
通过使用COMSOL进行模拟和优化,厂家成功地解决了元器件加热过程中的温度控制问题。
他们能够确保元器件在安全温度范围内进行加热,避免了因过高温度导致的损坏。
此外,优化后的加热过程还能够提高元器件的生产效率和质量,降低生产成本。
综上所述,COMSOL软件在电子元器件加热过程的优化中发挥了关键作用。
它通过建立和求解多物理场模型,帮助厂家实现了对加热过程的精确控制,提高了产品的质量和性能。
COMSOL3.5结构力学模型案例01

结构力学: 结构力学模型案例结构力学模型案例通过以下两个不同情况来介绍如何进行线性静态应力分析。
• 外边界的均布水平载荷• 重力载荷这个案例来自NAFEMS 基本系列 (参考文献. 1).锥形膜末端载荷第一个案例介绍厚度为0.1mm的膜的2D平面应力。
水平载荷沿右末端平均分布,为10 MN/m (也就是应力为 100 MPa)。
在左末端,x方向位移零。
左端的中间点固定在y方向。
模型使用以下材料属性:• 材料是各向同性的。
• 杨氏模量(弹性模量)为210·103 MPa。
• 泊松比为0.3。
在COMSOL Multiphysics中建模使用平面应力模式的静态分析,这样可以直接进行应力分析。
有限元模型使用拉格朗日二次三角单元。
为了确定结果已经收敛到基准值,细化网格然后再次计算结果。
结果点(0,2)处x方向应力求解值和基准目标值61.3 MPa吻合很好。
如果采用初始化网格,COMSOL Multiphysics 计算结果为61.41 MPa。
两次连续的细化网格后计算值分别为T 61.36 MPa 和 61.35 MPa。
图8-1: 均布末端载荷下x方向的应力分布模型库路径: COMSOL_Multiphysics/Structural_Mechanics/edge_load_2d 图形用户界面建模建模导航1 在空间维度下拉框中选择2D。
2 在应用模式树下,依次选择COMSOL Multiphysics>结构力学>平面应力>静态分析。
3 点击确定。
几何建模1 在绘图菜单下,选择指定对象>线。
2 在线对话框中,在x编辑框中输入0 4 4 0 0,在y编辑框中输入 0 134 0。
3 点击确定。
4 点击主工具栏的缩放至窗口大小按钮。
5 点击绘图工具栏的强迫成实体按钮。
定义的点就是约束点,也是应力基准值点。
物理量设定边界和点条件—载荷和约束求解域设定—材料属性6 在绘图菜单下,选择指定对象>点。
COMSOL案例详解 液滴models.mfl.droplet_breakup(优选.)
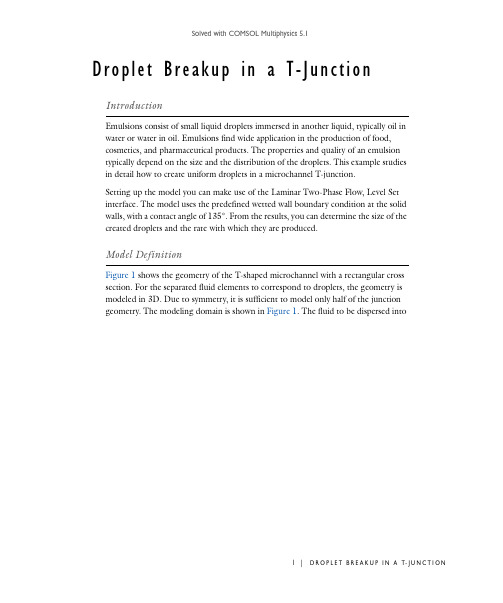
Droplet Breakup in a T-Junction IntroductionEmulsions consist of small liquid droplets immersed in another liquid, typically oil in water or water in oil. Emulsions find wide application in the production of food, cosmetics, and pharmaceutical products. The properties and quality of an emulsion typically depend on the size and the distribution of the droplets. This example studies in detail how to create uniform droplets in a microchannel T-junction.Setting up the model you can make use of the Laminar Two-Phase Flow, Level Set interface. The model uses the predefined wetted wall boundary condition at the solid walls, with a contact angle of 135°. From the results, you can determine the size of the created droplets and the rate with which they are produced.Model DefinitionFigure 1 shows the geometry of the T-shaped microchannel with a rectangular cross section. For the separated fluid elements to correspond to droplets, the geometry is modeled in 3D. Due to symmetry, it is sufficient to model only half of the junction geometry. The modeling domain is shown in Figure 1. The fluid to be dispersed intosmall droplets, Fluid 2, enters through the vertical channel. The other fluid, Fluid 1, flows from the right to left through the horizontal channel.Inlet, fluid 2Inlet, fluid 1Initial fluid interface OutletFigure 1: The modeling domain of the T-junction.The problem described is straight forward to set up with the Laminar Two-Phase Flow, Level Set interface. The interface sets up a momentum transport equation, a continuity equation, and a level set equation for the level set variable. The fluid interface is defined by the 0.5 contour of the level set function.The interface uses the following equations:In the equations above, ρ denotes density (kg/m 3), u velocity (m/s), t time (s), μ dynamic viscosity (Pa·s), p pressure (Pa), and F st the surface tension force (N/m 3). Furthermore, φ is the level set function, and γ and ε are numerical stabilization parameters. The density and viscosity are calculated fromρt∂∂u ρu ∇⋅()u +∇p –I μ∇u ∇u ()T +()+[]F st +⋅=∇u ⋅0=∂φ∂t -----u ∇φ⋅+γ∇φ–1φ–()∇φ∇φ----------ε∇φ+⎝⎭⎛⎫⋅=where ρ1, ρ2, μ1, and μ2 are the densities and viscosities of Fluid 1 and Fluid 2.P H Y S I C A L P A R A M E T E R SThe two liquids have the following physical properties:QUANTITY VALUE, FLUID 1VALUE, FLUID 2Density (kg/m 3)10001000Dynamic viscosity (Pa·s)0.001950.00671The surface tension coefficient is 5·10−3 N/m.B O U N D A R YC O ND I T I O N SAt both inlets, Laminar inflow conditions with prescribed volume flows are used. At the outflow boundary, the Pressure, no viscous stress condition is set. The Wetted wall boundary condition applies to all solid boundaries with the contact angle specified as 135° and a slip length equal to the mesh size parameter, h . The contact angle is the angle between the fluid interface and the solid wall at points where the fluid interface attaches to the wall. The slip length is the distance to the position outside the wall where the extrapolated tangential velocity component is zero (see Figure 2).Fluid 1Fluid 2Wall θWallβu Figure 2: The contact angle, θ, and the slip length, β.ρρ1ρ2ρ1–()φ+=μμ1μ2μ1–()φ+=Results and DiscussionFigure 3 shows the fluid interface (the level set function φ0.5=) and velocitystreamlines at various times. The first droplet is formed after approximately0.03 s.Figure 3: Velocity streamlines, velocity on the symmetry plane, and the phase boundary at t = 0.02 s, 0.04 s, 0.06 s, and 0.08 s.You can calculate the effective diameter, d eff —that is, the diameter of a sphericaldroplet with the same volume as the formed droplet—using the following expression:(1)Here, Ω represents the leftmost part of the horizontal channel, where x < −0.2 mm. In this case, the results show that d eff is about 0.12 mm. The results are in fair agreement with those presented in Ref. 1.d eff 234π------φ0.5>()Ωd Ω 3⋅=Reference1. S. van der Graaf, T. Nisisako, C. G. P. H. Schroën, R. G. M. van der Sman, and R. M. Boom, “Lattice Boltzmann Simulations of Droplet Formation in a T-Shaped Microchannel,” Langmuir, vol. 22, pp. 4144–4152, 2006.Application Library path: Microfluidics_Module/Two-Phase_Flow/droplet_breakupModeling InstructionsFrom the File menu, choose New.N E W1In the New window, click Model Wizard.M O D E L W I Z A R D1In the Model Wizard window, click 3D.2In the Select physics tree, select Fluid Flow>Multiphase Flow>Two-Phase Flow, Level Set>Laminar Two-Phase Flow, Level Set (tpf).3Click Add.4Click Study.5In the Select study tree, select Preset Studies>Transient with Phase Initialization.6Click Done.G E O M E T R Y11In the Model Builder window, under Component 1 (comp1) click Geometry 1.2In the Settings window for Geometry, locate the Units section.3From the Length unit list, choose mm.Work Plane 1 (wp1)1On the Geometry toolbar, click Work Plane.2In the Settings window for Work Plane, locate the Plane Definition section.3From the Plane list, choose xz-plane.Rectangle 1 (r1)1On the Geometry toolbar, click Primitives and choose Rectangle.2In the Settings window for Rectangle, locate the Size section.3In the Width text field, type 0.1.4In the Height text field, type 0.4.5Locate the Position section. In the yw text field, type 0.1.Rectangle 2 (r2)1On the Geometry toolbar, click Primitives and choose Rectangle.2In the Settings window for Rectangle, locate the Size section.3In the Width text field, type 1.4In the Height text field, type 0.1.5Locate the Position section. In the xw text field, type -0.7.Plane Geometry1On the Geometry toolbar, click Build All.2Click the Zoom Extents button on the Graphics toolbar.Polygon 1 (pol1)1On the Geometry toolbar, click Primitives and choose Polygon.2In the Settings window for Polygon, locate the Object Type section.3From the Type list, choose Open curve.4Locate the Coordinates section. In the xw text field, type 0 0.1.5In the yw text field, type 0.2 0.2.Plane GeometryRight-click Component 1 (comp1)>Geometry 1>Work Plane 1 (wp1)>Plane Geometry>Polygon 1 (pol1) and choose Build Selected.Polygon 2 (pol2)1On the Geometry toolbar, click Primitives and choose Polygon.2In the Settings window for Polygon, locate the Coordinates section.3In the xw text field, type 0.1 0.1.4In the yw text field, type 0 0.1.Work Plane 1 (wp1)Right-click Component 1 (comp1)>Geometry 1>Work Plane 1 (wp1)>PlaneGeometry>Polygon 2 (pol2) and choose Build Selected .Extrude 1 (ext1)1On the Geometry toolbar, click Extrude .2In the Settings window for Extrude, locate the Distances from Plane section.3In the table, enter the following settings:4Right-click Component 1 (comp1)>Geometry 1>Extrude 1 (ext1) and choose Build Selected .5Click the Zoom Extents button on the Graphics toolbar.Form Union (fin)1In the Model Builder window, under Component 1 (comp1)>Geometry 1 right-click Form Union (fin) and choose Build Selected . The geometry should look like in Figure 1.M A T E R I A L SMaterial 1 (mat1)1In the Model Builder window, under Component 1 (comp1) right-click Materials and choose Blank Material .2Right-click Material 1 (mat1) and choose Rename .3In the Rename Material dialog box, type Fluid 1 in the New label text field.4Click OK .5In the Settings window for Material, locate the Material Contents section.6In the table, enter the following settings:Distances (mm)0.05Property Name ValueUnit Property group Density rho 1e3[kg/m^3]kg/m³Basic Dynamic viscosity mu 1.95e-3[Pa*s]Pa·s BasicMaterial 2 (mat2)1In the Model Builder window, right-click Materials and choose Blank Material .2Right-click Material 2 (mat2) and choose Rename .3In the Rename Material dialog box, type Fluid 2 in the New label text field.4Click OK .5In the Settings window for Material, click to expand the Material properties section.6Locate the Material Properties section. In the Material properties tree, select Basic Properties>Density .7Click Add to Material .8In the Material properties tree, select Basic Properties>Dynamic Viscosity .9Click Add to Material .10Locate the Material Contents section. In the table, enter the following settings:D E F I N I T I O N SStep 1 (step1)1On the Home toolbar, click Functions and choose Local>Step .2In the Settings window for Step, locate the Parameters section.3In the Location text field, type 1e-3.4Click to expand the Smoothing section. In the Size of transition zone text field, type 2e-3.Add an integration operator that you will use to calculate the effective droplet diameter according to Equation 1 in the Model Definition section.Integration 1 (intop1)1On the Definitions toolbar, click Component Couplings and choose Integration .2In the Settings window for Integration, locate the Source Selection section.3From the Selection list, choose All domains .Property Name ValueUnit Property group Density rho 1e3[kg/m^3]kg/m³Basic Dynamic viscosity mu 6.71e-3[Pa*s]Pa·s BasicVariables 11On the Definitions toolbar, click Local Variables .2In the Settings window for Variables, locate the Variables section.3In the table, enter the following settings:L A M I N A R T W O -P H A S E F L O W, L E V E L S E T (T P F )The mesh can be controlled very well in this model, which makes it possible to use a lower element order without reducing the accuracy.1In the Model Builder window’s toolbar, click the Show button and select Discretization in the menu.2In the Model Builder window, expand the Component 1 (comp1)>Laminar Two-Phase Flow, Level Set (tpf) node, then click Laminar Two-Phase Flow, Level Set (tpf).3In the Settings window for Laminar Two-Phase Flow, Level Set, click to expand the Discretization section.4From the Discretization of fluids list, choose P1 + P1.Fluid Properties 11In the Model Builder window, under Component 1 (comp1)>Laminar Two-Phase Flow, Level Set (tpf) click Fluid Properties 1.2In the Settings window for Fluid Properties, locate the Fluid 1 Properties section.3From the Fluid 1 list, choose Fluid 1 (mat1).4Locate the Fluid 2 Properties section. From the Fluid 2 list, choose Fluid 2 (mat2).5Locate the Surface Tension section. From the Surface tension coefficient list, choose User defined . In the σ text field, type 5e-3[N/m].6Locate the Level Set Parameters section. In the γ text field, type 0.05[m/s].7In the εls text field, type 5e-6[m].NameExpression Unit Description V10.4e-6/3600*step1(t[1/s])[m^3/s]m³/s Volume flow, inlet 1V20.2e-6/3600*step1(t[1/s])[m^3/s]m³/s Volume flow, inlet 2d_eff 2*(intop1((phils>0.5)*(x<-0.2[mm]))*3/(4*pi))^(1/3)m Effective droplet diameterWall 1Because this is the default boundary condition node, you cannot modify the selection explicitly. Instead, you override the default condition where it is not applicable by adding other boundary conditions.1In the Model Builder window, under Component 1 (comp1)>Laminar Two-Phase Flow, Level Set (tpf) click Wall 1.2In the Settings window for Wall, locate the Boundary Condition section.3From the Boundary condition list, choose Wetted wall.4In the θw text field, type 3*pi/4[rad].5In the β text field, type 5e-6[m].Initial Interface 11In the Model Builder window, under Component 1 (comp1)>Laminar Two-Phase Flow, Level Set (tpf) click Initial Interface 1.2Select Boundary 11 only.Initial Values 21On the Physics toolbar, click Domains and choose Initial Values.2Select Domain 3 only.3In the Settings window for Initial Values, locate the Initial Values section.4From the Fluid initially in domain list, choose Fluid 2.For Domains 1 and 2, the default initial value settings apply.Inlet 11On the Physics toolbar, click Boundaries and choose Inlet.2Select Boundary 22 only.3In the Settings window for Inlet, locate the Boundary Condition section.4From the list, choose Laminar inflow.5Locate the Laminar Inflow section. Click the Flow rate button.6In the V0 text field, type V1.7In the L entr text field, type 0.01[m].Inlet 21On the Physics toolbar, click Boundaries and choose Inlet.2Select Boundary 12 only.3In the Settings window for Inlet, locate the Level Set Condition section.4In the V f text field, type 1.5Locate the Boundary Condition section. From the list, choose Laminar inflow.6Locate the Laminar Inflow section. Click the Flow rate button.7In the V0 text field, type V2.8In the L entr text field, type 0.01[m].Outlet 11On the Physics toolbar, click Boundaries and choose Outlet.2Select Boundary 1 only.Symmetry 11On the Physics toolbar, click Boundaries and choose Symmetry.2Select Boundaries 5, 13, 14, and 21 only.M E S H1Mapped 11In the Model Builder window, under Component 1 (comp1) right-click Mesh 1 andchoose More Operations>Mapped.2Select Boundaries 2, 7, 10, and 16 only.Distribution 11Right-click Component 1 (comp1)>Mesh 1>Mapped 1 and choose Distribution.2Select Edge 3 only.3In the Settings window for Distribution, locate the Distribution section.4In the Number of elements text field, type 160.Distribution 21Right-click Mapped 1 and choose Distribution.2Select Edges 1 and 9 only.3In the Settings window for Distribution, locate the Distribution section.4In the Number of elements text field, type 20.Distribution 31Right-click Mapped 1 and choose Distribution.2Select Edges 12 and 28 only.3In the Settings window for Distribution, locate the Distribution section.11|D R O P L E T B R E A K U P I N A T-J U N C T I O N4From the Distribution properties list, choose Predefined distribution type.5In the Number of elements text field, type 25.6In the Element ratio text field, type 4.Distribution 41Right-click Mapped 1 and choose Distribution.2Select Edges 24 and 27 only.3In the Settings window for Distribution, locate the Distribution section.4From the Distribution properties list, choose Predefined distribution type.5In the Number of elements text field, type 20.6In the Element ratio text field, type 3.7Select the Reverse direction check box.Mapped 1Right-click Mapped 1 and choose Build Selected.Swept 11Right-click Mesh 1 and choose Swept.2In the Settings window for Swept, click to expand the Source faces section.3Locate the Source Faces section. Select the Active toggle button.4Select Boundaries 2, 7, and 10 only.Distribution 11Right-click Component 1 (comp1)>Mesh 1>Swept 1 and choose Distribution.2In the Settings window for Distribution, locate the Distribution section.3In the Number of elements text field, type 10.12|D R O P L E T B R E A K U P I N A T-J U N C T I O N4Click the Build All button.S T U D Y1Step 2: Time Dependent1In the Model Builder window, expand the Study 1 node, then click Step 2: Time Dependent.2In the Settings window for Time Dependent, locate the Study Settings section.3In the Times text field, type range(0,5e-3,0.08).4Click to expand the Results while solving section. Locate the Results While Solvingsection. Select the Plot check box.5From the Plot group list, choose Default.This choice means that the Graphics window will show a surface plot of the volumefraction of Fluid 1 while solving, and this plot will be updated at each 5 ms output timestep.Manually tune the solver sequence for optimal performance and accuracy.Solution 11On the Study toolbar, click Show Default Solver.2In the Model Builder window, expand the Solution 1 node, then click Time-Dependent Solver 1.13|D R O P L E T B R E A K U P I N A T-J U N C T I O N3In the Settings window for Time-Dependent Solver, click to expand the Time stepping section.4Locate the Time Stepping section. From the Method list, choose Generalized alpha.5Select the Time step increase delay check box.6In the associated text field, type 3.7In the Amplification for high frequency text field, type 0.3.8From the Predictor list, choose Constant.9In the Model Builder window, expand the Study 1>Solver Configurations>Solution1>Time-Dependent Solver 1 node.10Right-click Study 1>Solver Configurations>Solution 1>Time-Dependent Solver 1 andchoose Iterative.11In the Settings window for Iterative, locate the Error section.12In the Factor in error estimate text field, type 20.13In the Maximum number of iterations text field, type 200.14Right-click Study 1>Solver Configurations>Solution 1>Time-Dependent Solver1>Iterative 1 and choose Multigrid.15In the Model Builder window, expand the Study 1>Solver Configurations>Solution1>Time-Dependent Solver 1>Iterative 1>Multigrid 1 node.16Right-click Presmoother and choose SCGS.17In the Settings window for SCGS, locate the Main section.18Select the Vanka check box.19Under Variables, click Add.20In the Add dialog box, In the Variables list, choose comp1.tpf.Pinlinl1 andcomp1.tpf.Pinlinl2.21Click OK.22In the Model Builder window, expand the Study 1>Solver Configurations>Solution1>Time-Dependent Solver 1>Iterative 1>Multigrid 1>Postsmoother node.23Right-click Study 1>Solver Configurations>Solution 1>Time-Dependent Solver1>Iterative 1>Multigrid 1>Postsmoother and choose SCGS.24In the Settings window for SCGS, locate the Main section.25Select the Vanka check box.26Under Variables, click Add.14|D R O P L E T B R E A K U P I N A T-J U N C T I O N27In the Add dialog box, In the Variables list, choose comp1.tpf.Pinlinl1 and comp1.tpf.Pinlinl2.28Click OK.29In the Model Builder window, expand the Study 1>Solver Configurations>Solution1>Time-Dependent Solver 1>Iterative 1>Multigrid 1>Coarse Solver node, then clickDirect.30In the Settings window for Direct, locate the General section.31From the Solver list, choose PARDISO.32In the Model Builder window, collapse the Study 1>Solver Configurations>Solution1>Time-Dependent Solver 1 node.S T U D Y1Solution 11In the Model Builder window, collapse the Study 1>Solver Configurations>Solution1>Time-Dependent Solver 1 node.2In the Model Builder window, collapse the Solution 1 node.3On the Study toolbar, click Compute.4Click the Zoom Extents button on the Graphics toolbar.R E S U L T SThe first default plot group shows the volume fraction of fluid 1 as slice plot, and thesecond plot group shows a slice plot of the velocity combined with a contour plot ofthe volume fraction of fluid 1. Follow these steps to reproduce the series of velocityfield plots shown in Figure 3.3D Plot Group 31On the Home toolbar, click Add Plot Group and choose 3D Plot Group.2In the Model Builder window, under Results right-click 3D Plot Group 3 and choose Slice.3In the Settings window for Slice, click Replace Expression in the upper-right cornerof the Expression section. From the menu, choose Component 1>Laminar Two-PhaseFlow, Level Set>tpf.U - Velocity magnitude.4Locate the Plane Data section. From the Plane list, choose zx-planes.5From the Entry method list, choose Coordinates.6On the 3D Plot Group 3 toolbar, click Plot.15|D R O P L E T B R E A K U P I N A T-J U N C T I O N7In the Model Builder window, right-click 3D Plot Group 3 and choose Isosurface.8In the Settings window for Isosurface, locate the Levels section.9From the Entry method list, choose Levels.10In the Levels text field, type 0.5.11Locate the Coloring and Style section. From the Coloring list, choose Uniform.12From the Color list, choose Green.13Right-click 3D Plot Group 3 and choose Streamline.14In the Settings window for Streamline, locate the Streamline Positioning section.15From the Positioning list, choose Uniform density.16In the Separating distance text field, type 0.05.17Locate the Coloring and Style section. From the Line type list, choose Tube.18Select the Radius scale factor check box.19In the associated text field, type 2e-3.20From the Color list, choose Yellow.21In the Model Builder window, click 3D Plot Group 3.22In the Settings window for 3D Plot Group, locate the Data section.23From the Time (s) list, choose 0.02.24On the 3D Plot Group 3 toolbar, click Plot.25Click the Go to Default 3D View button on the Graphics toolbar.Compare the resulting plot with the upper-left plot in Figure 3.To reproduce the remaining three plots, plot the solution for the time values 0.04,0.06, and 0.08 s.Next, evaluate the effective droplet diameter computed according to Equation 1.Derived Values1On the Results toolbar, click Global Evaluation.2In the Settings window for Global Evaluation, click Replace Expression in theupper-right corner of the Expression section. From the menu, choose Component1>Definitions>Variables>d_eff - Effective droplet diameter.3Click the Evaluate button.16|D R O P L E T B R E A K U P I N A T-J U N C T I O NT A B L E1Go to the Table window.The result, roughly 0.12 mm, is displayed in the table in the Table window.Finally, generate a movie of the moving fluid interface and the velocity streamlines.R E S U L T SExport1In the Model Builder window, under Results right-click 3D Plot Group 3 and choose Player.COMSOL Multiphysics generates the movie and then plays it.To replay the movie, click the Play button on the Graphics toolbar.If you want to export a movie in GIF, Flash, or AVI format, right-click Export andcreate an Animation feature.17|D R O P L E T B R E A K U P I N A T-J U N C T I O N18|D R O P L E T B R E A K U P I N A T-J U N C T I O N。
COMSOL案例详解 单相扩散 英文models.mfl.controlled_diffusion_micromixer
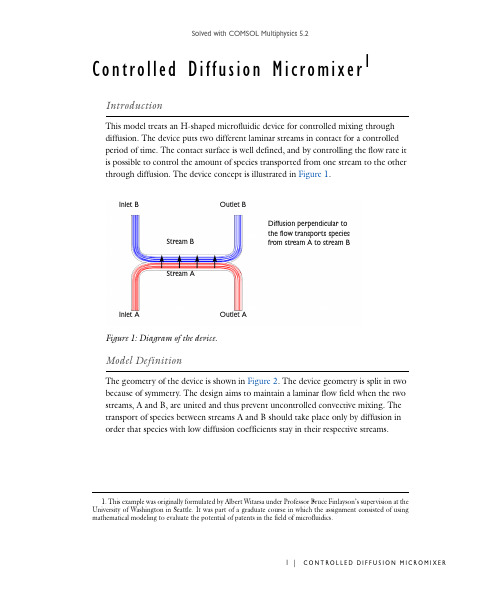
Controlled Diffusion Micromixer 1IntroductionThis model treats an H-shaped microfluidic device for controlled mixing through diffusion. The device puts two different laminar streams in contact for a controlled period of time. The contact surface is well defined, and by controlling the flow rate it is possible to control the amount of species transported from one stream to the other through diffusion. The device concept is illustrated in Figure 1.Figure 1: Diagram of the device.Model DefinitionThe geometry of the device is shown in Figure 2. The device geometry is split in two because of symmetry. The design aims to maintain a laminar flow field when the two streams, A and B, are united and thus prevent uncontrolled convective mixing. The transport of species between streams A and B should take place only by diffusion in order that species with low diffusion coefficients stay in their respective streams.1. This example was originally formulated by Albert Witarsa under Professor Bruce Finlayson’s supervision at the University of Washington in Seattle. It was part of a graduate course in which the assignment consisted of using mathematical modeling to evaluate the potential of patents in the field of microfluidics.Diffusion perpendicular tothe flow transports speciesfrom stream A to stream B Outlet AInlet A Stream AOutlet BInlet B Stream BFigure 2: Model geometry. To avoid any type of convective mixing, the design must smoothly let both streams come in contact with each other. Due to symmetry, it is sufficient to model half the geometry, so the actual channel is twice as high in the z-direction.The flow rate at the inlet is approximately 0.1 mm/s. The Reynolds number, which is important for characterizing the flow is given by:where ρ is the fluid density (1000 kg/m 3), U is a characteristic velocity of the flow (0.1 mm/s), μ is the fluid viscosity (1 mPa ⋅s) and L is a characteristic dimension of the device (10 μm). When the Reynolds number is significantly less than 1, as in this example, the Creeping Flow interface can be used. The convective term in theNavier-Stokes equations can be dropped, leaving the incompressible Stokes equations:where u is the local velocity (m/s) and p is the pressure (Pa).Mixing in the device involves species at relatively low concentrations compared to the solvent, in this case water. This means that the solute molecules interact only with Symmetry plane (blue)Re ρUL μ------------0,001==∇p I –μu ∇u ∇()T+()+()⋅0=∇u ⋅0=water molecules, and Fick’s law can be used to describe the diffusive transport. The mass-balance equation for the solute is therefore:where D is the diffusion coefficient of the solute (m 2/s) and c is its concentration (mol/m 3). Diffusive flows can be characterized by another dimensionless number: the Peclet number, which is given by:In this model, the parametric solver is used to solve Equation 1 for three different species, each with different values of D: 1×10-11 m 2/s, 5×10-11 m 2/s, and1×10-10 m 2/s. These values of D correspond to Peclet numbers of 100, 20 and 10 respectively. Since these Peclet numbers are all greater than 1, implying a cell Peclet number significantly greater than 1, numerical stabilization is required when solving Fick’s equation. COMSOL automatically includes the stabilization by default, so no explicit settings are required.Two versions of the model are solved:•In the first version, it is assumed that a change in solute concentration does not influence the fluid’s density and viscosity. This implies that it is possible to first solve the Navier-Stokes equations and then solve the mass balance equation.•In the second version, the viscosity depends quadratically on the concentration:Here α is a constant of dimension m 6/(mol)2 and μ0 is the viscosity at zeroconcentration. Such a relationship between concentration and viscosity is usually observed in solutions of larger molecules.Results and DiscussionFigure 3 shows the velocity field for the case where viscosity is concentrationindependent. The flow is symmetric and is not influenced by the concentration field. Figure 4 shows the corresponding pressure distribution on the channel walls that∇D c ∇–c u +()⋅–0=Pe LU D--------=μμ01αc 2+()=results from the flow.Figure 3: Flow velocity field.Figure 4: Pressure distribution on the channel walls.Figure 5: Concentration distribution for a species with diffusivity 1·10-11 m2/s.Figure 6: Concentration distribution for a species with diffusivity 5·10-11 m2/s.Figure 7: Concentration distribution for a species with diffusivity 1·10-10 m2/s.coefficient.Figure 5, Figure 6 and Figure 7 show the species concentration for the each of the three diffusion coefficients. For the heaviest species, which has the smallest diffusivity, there is almost no significant mixing between streams A and B (Figure 5). For the lightest species, which has the largest diffusion coefficient, the mixing is almost perfect (Figure 7). Figure 8 shows how the mean concentration of the species at the outlet of stream B varies for the different species diffusion coefficients. The simulation clearly shows that the device could be used to separate lighter molecules from heavier ones. By placing a number of these devices in series, a high degree of separation could be obtained.In some cases, especially those involving solutions of macromolecules, the macromolecule concentration has a large influence on the liquid’s viscosity. In such situations, the Navier-Stokes and the convection-diffusion equations become coupled, and so they must be solved simultaneously. Figure 9, Figure 10, and Figure 11 show the results of such a simulation, in which the Navier Stokes equations are solved with a concentration dependent viscosity. In this case the species with for the species with diffusivity 5·10−11 m2/s. The velocity field is altered slightly by the concentration dependent viscosity (see Figure 12) but this has little effect on the mean stream A outlet concentration, which changes only slightly from 0.450 to 0.451. Much more serious is the effect of the non-uniform viscosity on the pressure distribution required to maintain the two streams at the same flow rate. A larger pressure is required at the inlet of stream B to drive the higher viscosity fluid through the system. This asymmetry in the pressure distribution makes placing several devices in series much more difficult.Figure 9: Velocity field. The viscosity varies with the concentration according toμ= μ0(1 + α c2) with α = 0.5 (m3/mol)2. It is difficult to see the differences between this figure and that in Figure 3, but careful inspection reveals a slight change in the velocityprofile. This is highlighted further in Figure 12.Figure 10: Pressure distribution. The viscosity varies with the concentration according to μ= μ0(1 + α c2) with α = 0.5 (m3/mol)2. This figure should be compared to Figure 4.There are significant differences between the two cases.Figure 11: Concentration distribution for the species with diffusivity 5·10-11 m2/s for the case where the fluid viscosity varies with concentration. This plot is very similar to the corresponding plot for an uncoupled flow, shown in Figure 6.Figure 12: Comparison of the velocity field for the uncoupled and coupled flow simulations showing the difference between the two cases. The coupled flow, in which the fluid viscosity is a function of concentration, is asymmetric.Application Library path: Microfluidics_Module/Micromixers/controlled_diffusion_micromixerModeling InstructionsFrom the File menu, choose New.N E W1In the New window, click Model Wizard.M O D E L W I Z A R D1In the Model Wizard window, click 3D.2In the Select physics tree, select Fluid Flow>Single-Phase Flow>Creeping Flow (spf).3Click Add.4In the Select physics tree, select Chemical Species Transport>Transport of Diluted Species (tds).5Click Add.6Click Study.7In the Select study tree, select Preset Studies for Selected Physics Interfaces>Stationary. 8Click Done.G E O M E T R Y1For many microfluidic devices it is convenient to specify the geometry dimensions using micrometers.1In the Model Builder window, under Component 1 (comp1) click Geometry 1.2In the Settings window for Geometry, locate the Units section.3From the Length unit list, choose µm.The geometry can be constructed by extruding a 2 dimensional workplane. First draw a top down view of the structure in the workplane.Work Plane 1 (wp1)On the Geometry toolbar, click Work Plane.Rectangle 1 (r1)1On the Work Plane toolbar, click Primitives and choose Rectangle.2In the Settings window for Rectangle, locate the Size and Shape section.3In the Width text field, type 140.4In the Height text field, type 60.5Right-click Rectangle 1 (r1) and choose Build Selected.Rectangle 2 (r2)1On the Work Plane toolbar, click Primitives and choose Rectangle.2In the Settings window for Rectangle, locate the Size and Shape section.3In the Width text field, type 120.4In the Height text field, type 50.5Locate the Position section. In the xw text field, type 10.6In the yw text field, type 10.7Right-click Rectangle 2 (r2) and choose Build Selected.Difference 1 (dif1)1On the Work Plane toolbar, click Booleans and Partitions and choose Difference. 2Select the object r1 only.3In the Settings window for Difference, locate the Difference section.4Find the Objects to subtract subsection. Select the Active toggle button.5Select the object r2 only.6Right-click Difference 1 (dif1) and choose Build Selected.Fillet 1 (fil1)1On the Work Plane toolbar, click Fillet.2On the object dif1, select Points 3 and 5 only.3In the Settings window for Fillet, locate the Radius section.4In the Radius text field, type 10.5Right-click Fillet 1 (fil1) and choose Build Selected.Fillet 2 (fil2)1On the Work Plane toolbar, click Fillet.2On the object fil1, select Points 1 and 9 only.3In the Settings window for Fillet, locate the Radius section.4In the Radius text field, type 20.5Right-click Fillet 2 (fil2) and choose Build Selected.Mirror 1 (mir1)1On the Work Plane toolbar, click Transforms and choose Mirror.2Select the object fil2 only.3In the Settings window for Mirror, locate the Input section.4Select the Keep input objects check box.5Locate the Normal Vector to Line of Reflection section. In the xw text field, type 0. 6In the yw text field, type 1.7Right-click Mirror 1 (mir1) and choose Build Selected.Union 1 (uni1)1On the Work Plane toolbar, click Booleans and Partitions and choose Union.2Select the objects mir1 and fil2 only.3In the Settings window for Union, locate the Union section.4Clear the Keep interior boundaries check box.5Right-click Union 1 (uni1) and choose Build Selected.Extrude the 2D geometry to create a 3 dimensional geometry.Extrude 1 (ext1)1On the Geometry toolbar, click Extrude.2In the Settings window for Extrude, locate the Distances from Plane section.3In the table, enter the following settings:Distances (µm)104Right-click Extrude 1 (ext1) and choose Build Selected.5Click the Zoom Extents button on the Graphics toolbar.Create parameters to define the model.G L O B A L D E F I N I T I O N SParameters1On the Home toolbar, click Parameters .2In the Settings window for Parameters, locate the Parameters section.3In the table, enter the following settings:Create an average operator to compute the mean output Expression ValueDescription D5e-11[m^2/s]5E-11 m²/s Diffusion constant fr15[pl/s] 1.5E-14 m³/s Inlet flow rate c01[mol/m^3] 1 mol/m³Inlet concentration alpha 0.5[(m^3/mol)^2]0.5 m^6/mol²Viscosity c^2-term prefactorD E F I N I T I O N SAverage 1 (aveop1)1On the Definitions toolbar, click Component Couplings and choose Average.2In the Settings window for Average, locate the Source Selection section.3From the Geometric entity level list, choose Boundary.4Select Boundary 22 only.Note that there are several methods to select a geometric entity. To select, for example, a boundary, place the cursor over the boundary in the graphics window. If the required boundary is not highlighted in red then rotate the scroll wheel until it is highlighted, then left click to select it (the up and down arrow keys can also be used instead of the scroll wheel). Alternatively, use the Selection list to display a list of the available boundary numbers (available from the Home>More Windows menu if you are using windows, or from the Windows menu on other platforms). Boundaries are added to the selection by first left clicking on the boundary and then right clicking and selecting Add to selection. To add several boundaries, hold the Ctrl key down when left clicking.For more information about selecting geometric entities in the Graphics window, see Creating a Geometry for Analysis in the COMSOL Multiphysics Reference Manual.Add material properties to the model.M A T E R I A L SMaterial 1 (mat1)1In the Model Builder window, under Component 1 (comp1) right-click Materials and choose Blank Material.2In the Settings window for Material, locate the Material Contents section.3In the table, enter the following settings:Property Name Value Unit Property groupDensity rho1000kg/m³BasicDynamic viscosity mu1e-3Pa·s BasicC R E E P I N G F L O W(S P F)Note that several default nodes have been added automatically to the model tree. The ‘D’ in the upper left corner of the node indicates that a node has been added by default.Add a laminar flow inlet boundary condition.Inlet 11On the Physics toolbar, click Boundaries and choose Inlet.2Select Boundary 2 only.3In the Settings window for Inlet, locate the Boundary Condition section.4From the list, choose Laminar inflow.5Locate the Laminar Inflow section. Click the Flow rate button.6In the V0 text field, type fr/2.Set the flow rate to one half of the parameter value, since only half of the geometry is modeled.7In the L entr text field, type 100[um].The entrance length should be set to a value large enough for a laminar flow to develop.Inlet 21Right-click Inlet 1 and choose Duplicate.2In the Settings window for Inlet, locate the Boundary Selection section.3Click Clear Selection.4Select Boundary 8 only.Add an outlet with a pressure boundary condition.Outlet 11On the Physics toolbar, click Boundaries and choose Outlet.2Select Boundaries 20 and 22 only.The default pressure of 0 Pa is appropriate in this case.Add a symmetry boundary condition in the symmetry plane.Symmetry 11On the Physics toolbar, click Boundaries and choose Symmetry.2Select Boundary 4 only.Now set up the mass transport physics.T R A N S P O R T O F D I L U T E D S P E C I E S(T D S)Transport Properties 1Use the computed velocity field for the species convection.1In the Model Builder window, expand the Component 1 (comp1)>Transport of Diluted Species (tds) node, then click Transport Properties 1.2In the Settings window for Transport Properties, locate the Model Inputs section. 3From the u list, choose Velocity field (spf).The diffusion coefficient is set to use the parameter previously defined.4Locate the Diffusion section. In the D c text field, type D.Specify the concentration at the two inlets.5In the Model Builder window, click Transport of Diluted Species (tds).Concentration 11On the Physics toolbar, click Boundaries and choose Concentration.2Select Boundary 2 only.3In the Settings window for Concentration, locate the Concentration section.4Select the Species c check box.5In the c0,c text field, type c0.Concentration 21On the Physics toolbar, click Boundaries and choose Concentration.2Select Boundary 8 only.3In the Settings window for Concentration, locate the Concentration section.4Select the Species c check box.In this case the concentration should take the default value of 0.Use the outflow condition to allow species to leave the domain by convection.Outflow 11On the Physics toolbar, click Boundaries and choose Outflow.2In the Settings window for Outflow, locate the Boundary Selection section.3Click Paste Selection.4In the Paste Selection dialog box, type 20 22 in the Selection text field.5Click OK.Set up the mesh. Microfluidic devices are frequently extruded 2D geometries. It is therefore frequently convenient to extrude a 2D mesh. First mesh the top face of the device with a 2D quad mesh.M E S H1Free Quad 11In the Model Builder window, under Component 1 (comp1) right-click Mesh 1 and choose More Operations>Free Quad.2Select Boundary 4 only.Size1In the Model Builder window, under Component 1 (comp1)>Mesh 1 click Size.2In the Settings window for Size, locate the Element Size section.3From the Calibrate for list, choose Fluid dynamics.4From the Predefined list, choose Coarse.Next, sweep the mesh through the domain.Swept 11In the Model Builder window, right-click Mesh 1 and choose Swept.2Right-click Swept 1 and choose Build All.Now set up a study to solve the problem. Initially it is assumed that the fluid flow and diffusion problems are uncoupled. In this case it makes sense to solve the fluidflow problem first and then to use the velocity field as an input for the diffusion problem. This will save time and memory, particularly since the diffusion problem is solved for three parameters.S T U D Y1Since the study will automatically generate a large number of default plots, default plots are disabled.1In the Model Builder window, click Study 1.2In the Settings window for Study, locate the Study Settings section.3Clear the Generate default plots check box.For step 1, solve only the creeping flow problem.Step 1: Stationary1In the Model Builder window, under Study 1 click Step 1: Stationary.2In the Settings window for Stationary, locate the Physics and Variables Selection section.3In the table, enter the following settings:Physics interface Solve for DiscretizationT ransport of Diluted Species physicsAdd a second study step.Stationary 2On the Study toolbar, click Study Steps and choose Stationary>Stationary.Step 2: Stationary 2Disable the solution of the creeping flow problem for this step, but import the previously computed solution into the relevant dependent variables so that they can be used to compute the convective species transport.1In the Settings window for Stationary, locate the Physics and Variables Selection section.2In the table, enter the following settings:Physics interface Solve for DiscretizationCreeping Flow physics3Click to expand the Values of dependent variables section. Locate the Values of Dependent Variables section. Find the Values of variables not solved for subsection. From the Settings list, choose User controlled.4From the Method list, choose Solution.5From the Study list, choose Study 1, Stationary.Solve the diffusion problem for three values of the diffusion coefficient.6Click to expand the Study extensions section. Locate the Study Extensions section. Select the Auxiliary sweep check box.7Click Add.8In the table, enter the following settings:Parameter name Parameter value list Parameter unitD1e-10 5e-11 1e-119On the Study toolbar, click Compute.Create a surface data set to view the pressure on the channel walls.R E S U L T SSurface 1On the Results toolbar, click More Data Sets and choose Surface.Data Sets1Select Boundaries 1, 3, 5–7, 9–19, 21, 23, and 24 only.First plot the velocity of the flow within the channel.3D Plot Group 11On the Results toolbar, click 3D Plot Group.Use a slice plot to view the data on one or more slices through the geometry.2In the Model Builder window, right-click 3D Plot Group 1 and choose Slice.3In the Settings window for Slice, locate the Expression section.4From the Unit list, choose mm/s.Add additional slices in different directions.5Right-click 3D Plot Group 1 and choose Slice.6In the Settings window for Slice, locate the Expression section.7From the Unit list, choose mm/s.8Locate the Plane Data section. From the Plane list, choose xy-planes.9In the Planes text field, type 1.The extra slices should use the same scale and colors for the velocity plot as the existing slice.10Click to expand the Inherit style section. Locate the Inherit Style section. From the Plot list, choose Slice 1.To avoid duplicate titles, turn off the title for additional slices.11Click to expand the Title section. From the Title type list, choose None.Add slices in a third plane.12Right-click Results>3D Plot Group 1>Slice 2 and choose Duplicate.13In the Settings window for Slice, locate the Plane Data section.14From the Plane list, choose zx-planes.15In the Planes text field, type 2.Use the arrow volume plot to visualize the flow direction.16In the Model Builder window, right-click 3D Plot Group 1 and choose Arrow Volume.17In the Settings window for Arrow Volume, locate the Arrow Positioning section.18Find the x grid points subsection. In the Points text field, type 14.19Find the y grid points subsection. In the Points text field, type 21.20Find the z grid points subsection. In the Points text field, type 3.21Locate the Coloring and Style section. From the Color list, choose Black.Rename the plot.22Right-click 3D Plot Group 1 and choose Rename.23In the Rename 3D Plot Group dialog box, type Velocity (uncoupled flow) in the New label text field.24Click OK.25On the Velocity (uncoupled flow) toolbar, click Plot.Next add a pressure plot, using the data set created previously.3D Plot Group 21On the Home toolbar, click Add Plot Group and choose 3D Plot Group.2In the Settings window for 3D Plot Group, locate the Data section.3From the Data set list, choose Surface 1.First add a uniformly colored surface, to highlight the channel walls.4Right-click 3D Plot Group 2 and choose Surface.5In the Settings window for Surface, locate the Expression section.6In the Expression text field, type 1.7Locate the Coloring and Style section. From the Coloring list, choose Uniform. 8From the Color list, choose Gray.Next use contours to visualize the pressure.9Right-click 3D Plot Group 2 and choose Contour.10In the Settings window for Contour, click Replace Expression in the upper-right corner of the Expression section. From the menu, choose Model>Component1>Creeping Flow>Velocity and pressure>p - Pressure.Finally rename the plot.11Right-click 3D Plot Group 2 and choose Rename.12In the Rename 3D Plot Group dialog box, type Pressure (uncoupled flow) in the New label text field.13Click OK.14On the Pressure (uncoupled flow) toolbar, click Plot.Next create a slice plot to visualize the concentration in the device. Use the existing velocity slice plot as a basis for this plot.Velocity (uncoupled flow) 11In the Model Builder window, under Results right-click Velocity (uncoupled flow) and choose Duplicate.Re-name the plot.2In the Model Builder window, under Results right-click Velocity (uncoupled flow) 1 and choose Rename.3In the Rename 3D Plot Group dialog box, type Concentration (uncoupled flow) in the New label text field.4Click OK.For each of the slice sub-nodes, change the plotted quantity to concentration.Concentration (uncoupled flow)1In the Model Builder window, expand the Results>Concentration (uncoupled flow) node, then click Slice 1.2In the Settings window for Slice, click Replace Expression in the upper-right corner of the Expression section. From the menu, choose Model>Component 1>Transport of Diluted Species>c - Concentration.3In the Model Builder window, under Results>Concentration (uncoupled flow) click Slice 2.4In the Settings window for Slice, click Replace Expression in the upper-right corner of the Expression section. From the menu, choose c - Concentration.5In the Model Builder window, under Results>Concentration (uncoupled flow) click Slice 3.6In the Settings window for Slice, click Replace Expression in the upper-right corner of the Expression section. From the menu, choose c - Concentration.Disable the arrow volume plot.7In the Model Builder window, under Results>Concentration (uncoupled flow)right-click Arrow Volume 1 and choose Disable.8In the Model Builder window, click Concentration (uncoupled flow).9On the Concentration (uncoupled flow) toolbar, click Plot.Look at the plot for each of the three diffusion coefficient levels.The plots on the next page show the results for each of the diffusion coefficients solved for. For the heaviest species, which has the smallest diffusivity, there is limited mixing between streams A and B. For the lightest species, which has the largest diffusion coefficient, the mixing is almost perfect.10Click Plot.11In the Settings window for 3D Plot Group, locate the Data section. 12From the Parameter value (D) list, choose 5E-11.13On the Concentration (uncoupled flow) toolbar, click Plot.14From the Parameter value (D) list, choose 1E-10.15On the Concentration (uncoupled flow) toolbar, click Plot .Add a global plot to show how the concentration at the output differs with diffusion coefficient.1D Plot Group 4On the Home toolbar, click Add Plot Group and choose 1D Plot Group .Global 1On the 1D Plot Group 4 toolbar, click Global .1D Plot Group 4Use the previously defined average operator to compute the average concentration at the device output.1In the Settings window for Global, locate the y-Axis Data section.2In the table, enter the following settings:No legend is necessary for this plot, as only one quantity is plotted.3Click to expand the Legends section. Clear the Show legends check box.Add a marker in the computed data points.Expression UnitDescription aveop1(c)mol/m^34Click to expand the Coloring and style section. Locate the Coloring and Style section. Find the Line markers subsection. From the Marker list, choose Point.5From the Positioning list, choose In data points.Change the axes titles. Note that html tags and a range of mathematical symbols and greek letters can be entered in the axes and plot titles. Search for Using Special Formats and Symbols in Titles in the COMSOL Multiphysics Reference Manual.6In the Model Builder window, click 1D Plot Group 4.7In the Settings window for 1D Plot Group, locate the Plot Settings section.8Select the x-axis label check box.9In the associated text field, type Diffusion Coefficient (m<sup>2</sup>/s).10Select the y-axis label check box.11In the associated text field, type Concentration at Stream B Outlet (mol/ m<sup>3</sup>).Change the axis limits for the plot.12Click to expand the Axis section. Select the Manual axis limits check box.13In the y minimum text field, type 0.14In the y maximum text field, type 0.5.Finally re-name the plot.15Right-click 1D Plot Group 4 and choose Rename.16In the Rename 1D Plot Group dialog box, type Output Concentration (uncoupled flow) in the New label text field.17Click OK.18On the Output Concentration (uncoupled flow) toolbar, click Plot.This plot shows that the concentration of the species at the output is strongly dependent on the diffusion coefficient of the molecule. Thus the device could be used to separate species with different diffusion coefficients, particularly if multiple stages of the device were arranged in series.In some cases, particularly if the solution consists of large macromolecules, the dissolved species has a large influence on the liquid’s viscosity. In such situations, the。
comsol案例
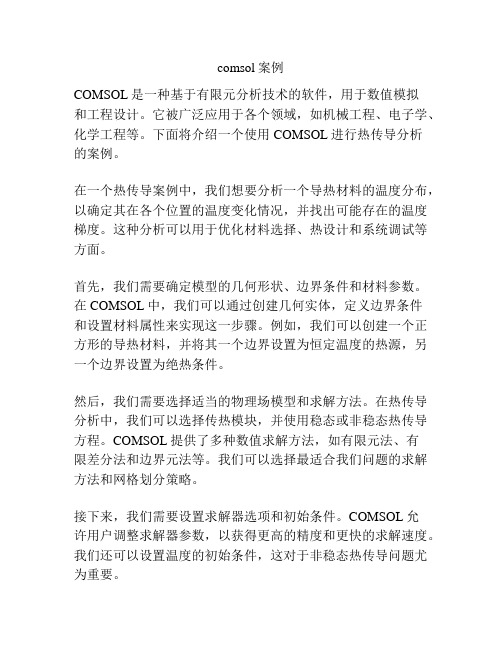
comsol案例COMSOL是一种基于有限元分析技术的软件,用于数值模拟和工程设计。
它被广泛应用于各个领域,如机械工程、电子学、化学工程等。
下面将介绍一个使用COMSOL进行热传导分析的案例。
在一个热传导案例中,我们想要分析一个导热材料的温度分布,以确定其在各个位置的温度变化情况,并找出可能存在的温度梯度。
这种分析可以用于优化材料选择、热设计和系统调试等方面。
首先,我们需要确定模型的几何形状、边界条件和材料参数。
在COMSOL中,我们可以通过创建几何实体,定义边界条件和设置材料属性来实现这一步骤。
例如,我们可以创建一个正方形的导热材料,并将其一个边界设置为恒定温度的热源,另一个边界设置为绝热条件。
然后,我们需要选择适当的物理场模型和求解方法。
在热传导分析中,我们可以选择传热模块,并使用稳态或非稳态热传导方程。
COMSOL提供了多种数值求解方法,如有限元法、有限差分法和边界元法等。
我们可以选择最适合我们问题的求解方法和网格划分策略。
接下来,我们需要设置求解器选项和初始条件。
COMSOL允许用户调整求解器参数,以获得更高的精度和更快的求解速度。
我们还可以设置温度的初始条件,这对于非稳态热传导问题尤为重要。
然后,我们可以进行计算并分析结果。
COMSOL提供了强大的后处理功能,可以用于可视化和分析模拟结果。
我们可以绘制温度分布图、温度剖面图,并计算温度梯度和导热通量等参数。
最后,我们可以根据分析结果来优化我们的设计。
例如,我们可以调整材料属性、改变几何形状或调整边界条件,以改善热传导性能或适应特定的设计要求。
综上所述,COMSOL提供了一种强大的工具,用于热传导分析和工程设计。
通过COMSOL的模拟和分析,工程师可以更好地理解热传导过程,并通过优化设计来改进性能和满足特定需求。
comsol 案例
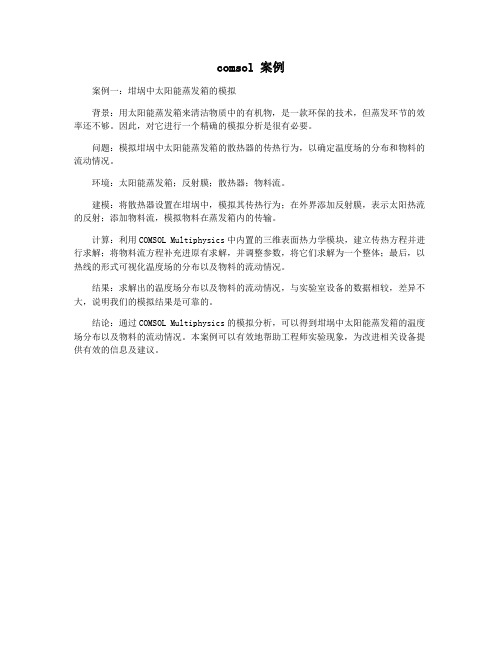
comsol 案例
案例一:坩埚中太阳能蒸发箱的模拟
背景:用太阳能蒸发箱来清洁物质中的有机物,是一款环保的技术,但蒸发环节的效率还不够。
因此,对它进行一个精确的模拟分析是很有必要。
问题:模拟坩埚中太阳能蒸发箱的散热器的传热行为,以确定温度场的分布和物料的流动情况。
环境:太阳能蒸发箱;反射膜;散热器;物料流。
建模:将散热器设置在坩埚中,模拟其传热行为;在外界添加反射膜,表示太阳热流的反射;添加物料流,模拟物料在蒸发箱内的传输。
计算:利用COMSOL Multiphysics中内置的三维表面热力学模块,建立传热方程并进行求解;将物料流方程补充进原有求解,并调整参数,将它们求解为一个整体;最后,以热线的形式可视化温度场的分布以及物料的流动情况。
结果:求解出的温度场分布以及物料的流动情况,与实验室设备的数据相较,差异不大,说明我们的模拟结果是可靠的。
结论:通过COMSOL Multiphysics的模拟分析,可以得到坩埚中太阳能蒸发箱的温度场分布以及物料的流动情况。
本案例可以有效地帮助工程师实验现象,为改进相关设备提供有效的信息及建议。
COMSOL官方实例解析
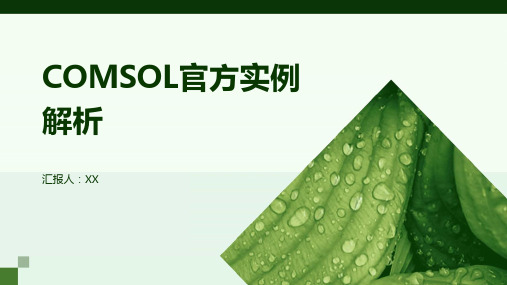
结果分析和讨论
01
结果展示
通过COMSOL的后处理功能,可以得到悬臂梁的变形图、应力分布图
等。
02 03
结果分析
从变形图可以看出,悬臂梁在受到集中载荷作用下发生了明显的弯曲变 形。从应力分布图可以看出,最大应力出现在固定端附近,且随着距离 的增加而逐渐减小。
讨论
在实际工程中,为了保证结构的安全性,需要对悬臂梁进行强度校核和 稳定性分析。此外,还可以通过优化设计方法,对悬臂梁的结构进行优 化,以减小变形和应力集中。
COMSOL官方实例 解析
汇报人:XX
目 录
• 引言 • 官方实例概述 • 实例解析:电磁场模拟 • 实例解析:结构力学分析 • 实例解析:流体动力学模拟 • 实例解析:传热模拟 • 总结与展望
01
引言
COMSOL软件简介
COMSOL是一款强大的多物理场仿真软件
它提供了广泛的物理接口和工具,用于模拟各种复杂的物理现象和工程问题。
要点二
边界条件
在平板的一侧施加恒定热流密度,例如1000 W/m²。另一 侧暴露在恒温环境中,例如25°C。平板的初始温度假设为 25°C。
结果分析和讨论
01 02 03
温度分布
通过求解模型,可以得到平板内的温度分布。可以观察到 ,在施加热流密度的一侧,温度迅速升高,而在暴露于恒 温环境的一侧,温度逐渐降低。温度梯度在平板内形成, 导致热量从高温区域向低温区域传递。
建模方法
使用COMSOL的传热模块进行建模。首先 ,定义几何形状和尺寸,然后选择合适的物 理场接口(如传热接口),并设置相应的材 料属性和边界条件。
材料属性和边界条件设置
要点一
材料属性
假设平板是由铜制成的,其热导率为385 W/(m·K),密度 为8960 kg/m³,比热容为385 J/(kg·K)。
Comsol经典实例013:射频消融、微波烧蚀肿瘤
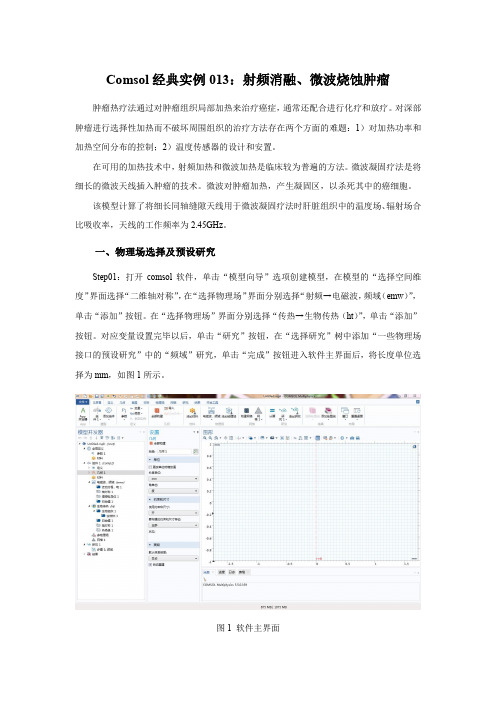
Comsol经典实例013:射频消融、微波烧蚀肿瘤肿瘤热疗法通过对肿瘤组织局部加热来治疗癌症,通常还配合进行化疗和放疗。
对深部肿瘤进行选择性加热而不破坏周围组织的治疗方法存在两个方面的难题:1)对加热功率和加热空间分布的控制;2)温度传感器的设计和安置。
在可用的加热技术中,射频加热和微波加热是临床较为普遍的方法。
微波凝固疗法是将细长的微波天线插入肿瘤的技术。
微波对肿瘤加热,产生凝固区,以杀死其中的癌细胞。
该模型计算了将细长同轴缝隙天线用于微波凝固疗法时肝脏组织中的温度场、辐射场合比吸收率,天线的工作频率为2.45GHz。
一、物理场选择及预设研究Step01:打开comsol软件,单击“模型向导”选项创建模型,在模型的“选择空间维度”界面选择“二维轴对称”,在“选择物理场”界面分别选择“射频→电磁波,频域(emw)”,单击“添加”按钮。
在“选择物理场”界面分别选择“传热→生物传热(ht)”,单击“添加”按钮。
对应变量设置完毕以后,单击“研究”按钮,在“选择研究”树中添加“一些物理场接口的预设研究”中的“频域”研究,单击“完成”按钮进入软件主界面后,将长度单位选择为mm,如图1所示。
图1 软件主界面二、几何模型1.绘制矩形Step02:右键单击“几何1”节点,在弹出的菜单中选择“矩形”,在“矩形”设置窗口,定位到“大小和形状”栏,在“宽度”文本输入框中输入40,在“高度”文本输入框中输入60。
单击“构建选定对象”,如图2所示。
图2 创建“矩形1”Step03:右键单击“几何1”节点,在弹出的菜单中选择“矩形”,在“矩形”设置窗口,定位到“大小和形状”栏,在“宽度”文本输入框中输入0.6,在“高度”文本输入框中输入50;定位到“位置”栏,在“z”文本输入框中输入10。
单击“构建选定对象”,如图3所示。
Step04:右键单击“几何1”节点,在弹出的菜单中选择“矩形”,在“矩形”设置窗口,在“标签”文本输入框中输入“介质层”;定位到“大小和形状”栏,在“宽度”文本输入框中输入0.34,在“高度”文本输入框中输入49.9;定位到“位置”栏,在“r”文本输入框中输入0.135,在“z”文本输入框中输入10.1。
comsol案例
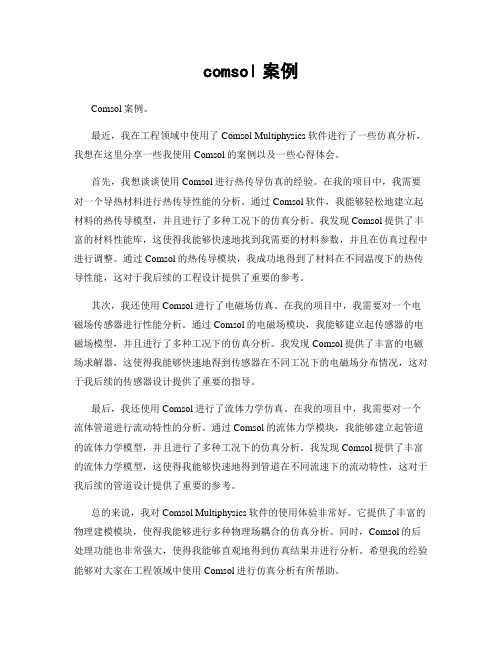
comsol案例Comsol案例。
最近,我在工程领域中使用了Comsol Multiphysics软件进行了一些仿真分析,我想在这里分享一些我使用Comsol的案例以及一些心得体会。
首先,我想谈谈使用Comsol进行热传导仿真的经验。
在我的项目中,我需要对一个导热材料进行热传导性能的分析。
通过Comsol软件,我能够轻松地建立起材料的热传导模型,并且进行了多种工况下的仿真分析。
我发现Comsol提供了丰富的材料性能库,这使得我能够快速地找到我需要的材料参数,并且在仿真过程中进行调整。
通过Comsol的热传导模块,我成功地得到了材料在不同温度下的热传导性能,这对于我后续的工程设计提供了重要的参考。
其次,我还使用Comsol进行了电磁场仿真。
在我的项目中,我需要对一个电磁场传感器进行性能分析。
通过Comsol的电磁场模块,我能够建立起传感器的电磁场模型,并且进行了多种工况下的仿真分析。
我发现Comsol提供了丰富的电磁场求解器,这使得我能够快速地得到传感器在不同工况下的电磁场分布情况,这对于我后续的传感器设计提供了重要的指导。
最后,我还使用Comsol进行了流体力学仿真。
在我的项目中,我需要对一个流体管道进行流动特性的分析。
通过Comsol的流体力学模块,我能够建立起管道的流体力学模型,并且进行了多种工况下的仿真分析。
我发现Comsol提供了丰富的流体力学模型,这使得我能够快速地得到管道在不同流速下的流动特性,这对于我后续的管道设计提供了重要的参考。
总的来说,我对Comsol Multiphysics软件的使用体验非常好。
它提供了丰富的物理建模模块,使得我能够进行多种物理场耦合的仿真分析。
同时,Comsol的后处理功能也非常强大,使得我能够直观地得到仿真结果并进行分析。
希望我的经验能够对大家在工程领域中使用Comsol进行仿真分析有所帮助。
comsol 案例

comsol 案例Comsol 案例。
在工程领域,计算机辅助工程仿真软件的应用越来越广泛。
COMSOL Multiphysics作为一款领先的多物理场仿真软件,被广泛应用于电磁、热传导、结构力学、流体力学等领域。
本文将介绍一个基于COMSOL Multiphysics的案例,以展示该软件在实际工程问题中的应用。
我们选取了一个热传导问题作为案例,以展示COMSOL Multiphysics在热传导领域的应用。
在这个案例中,我们需要分析一个复杂形状的导热体在不同热边界条件下的温度分布情况。
首先,我们需要建立该导热体的几何模型,然后设置热边界条件和材料属性,最后进行数值求解,得到温度场的分布情况。
在COMSOL Multiphysics中,建立几何模型可以通过几何建模模块来实现。
用户可以通过绘制几何形状、操作几何体等方式,快速建立复杂的几何模型。
在我们的案例中,我们需要考虑导热体的复杂形状,因此需要充分利用COMSOL Multiphysics提供的几何建模功能,精确地重现实际工程中的几何形状。
在几何模型建立完成后,我们需要设置热边界条件和材料属性。
COMSOL Multiphysics提供了丰富的物理场模块,用户可以根据实际问题选择相应的物理场模块进行建模。
在我们的案例中,我们需要选择热传导模块,然后设置热边界条件和材料属性。
COMSOL Multiphysics提供了直观的界面和丰富的选项,用户可以方便地设置各种热边界条件和材料属性,以满足实际工程问题的需求。
最后,我们进行数值求解,得到温度场的分布情况。
COMSOL Multiphysics采用有限元方法进行数值求解,能够精确地求解各种复杂的多物理场耦合问题。
在我们的案例中,通过COMSOL Multiphysics进行数值求解,我们可以得到导热体在不同热边界条件下的温度分布情况,从而为工程实践提供重要的参考。
通过上述案例,我们可以看到COMSOL Multiphysics在热传导领域的强大应用能力。
comsol 案例
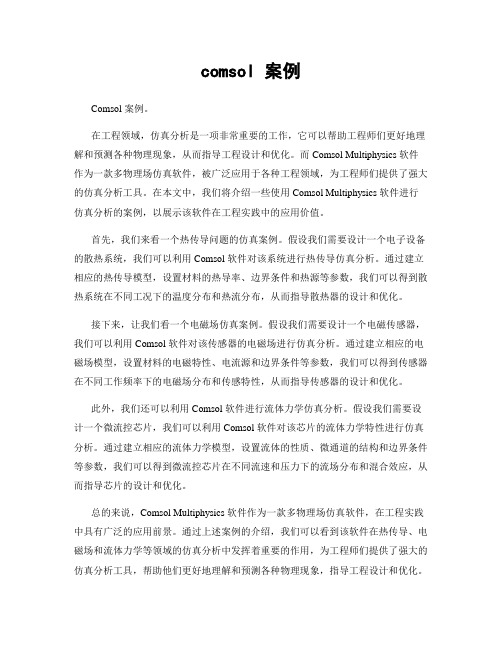
comsol 案例Comsol 案例。
在工程领域,仿真分析是一项非常重要的工作,它可以帮助工程师们更好地理解和预测各种物理现象,从而指导工程设计和优化。
而 Comsol Multiphysics 软件作为一款多物理场仿真软件,被广泛应用于各种工程领域,为工程师们提供了强大的仿真分析工具。
在本文中,我们将介绍一些使用 Comsol Multiphysics 软件进行仿真分析的案例,以展示该软件在工程实践中的应用价值。
首先,我们来看一个热传导问题的仿真案例。
假设我们需要设计一个电子设备的散热系统,我们可以利用 Comsol 软件对该系统进行热传导仿真分析。
通过建立相应的热传导模型,设置材料的热导率、边界条件和热源等参数,我们可以得到散热系统在不同工况下的温度分布和热流分布,从而指导散热器的设计和优化。
接下来,让我们看一个电磁场仿真案例。
假设我们需要设计一个电磁传感器,我们可以利用 Comsol 软件对该传感器的电磁场进行仿真分析。
通过建立相应的电磁场模型,设置材料的电磁特性、电流源和边界条件等参数,我们可以得到传感器在不同工作频率下的电磁场分布和传感特性,从而指导传感器的设计和优化。
此外,我们还可以利用 Comsol 软件进行流体力学仿真分析。
假设我们需要设计一个微流控芯片,我们可以利用 Comsol 软件对该芯片的流体力学特性进行仿真分析。
通过建立相应的流体力学模型,设置流体的性质、微通道的结构和边界条件等参数,我们可以得到微流控芯片在不同流速和压力下的流场分布和混合效应,从而指导芯片的设计和优化。
总的来说,Comsol Multiphysics 软件作为一款多物理场仿真软件,在工程实践中具有广泛的应用前景。
通过上述案例的介绍,我们可以看到该软件在热传导、电磁场和流体力学等领域的仿真分析中发挥着重要的作用,为工程师们提供了强大的仿真分析工具,帮助他们更好地理解和预测各种物理现象,指导工程设计和优化。
COMSOL案例详解 液滴models.mfl.droplet_breakup

Ω ( φ > 0.5 ) dΩ
(1)
Here, Ω represents the leftmost part of the horizontal channel, where x < −0.2 mm. In this case, the results show that deff is about 0.12 mm. The results are in fair agreement with those presented in Ref. 1.
Results and Discussion
Figure 3 shows the fluid interface (the level set function φ = 0.5 ) and velocity streamlines at various times. The first droplet is formed after approximately 0.03 s.
Solved with COMSOL Multiphysics 5.1
D r o ple t Br e a k up i n a T- Ju n ct i on
Introduction
Emulsions consist of small liquid droplets immersed in another liquid, typically oil in water or water in oil. Emulsions find wide application in the production of food, cosmetics, and pharmaceutical products. The properties and quality of an emulsion typically depend on the size and the distribution of the droplets. This example studies in detail how to create uniform droplets in a microchannel T-junction. Setting up the model you can make use of the Laminar Two-Phase Flow, Level Set interface. The model uses the predefined wetted wall boundary condition at the solid walls, with a contact angle of 135°. From the results, you can determine the size of the created droplets and the rate with which they are produced.
COMSOL案例electricsensor
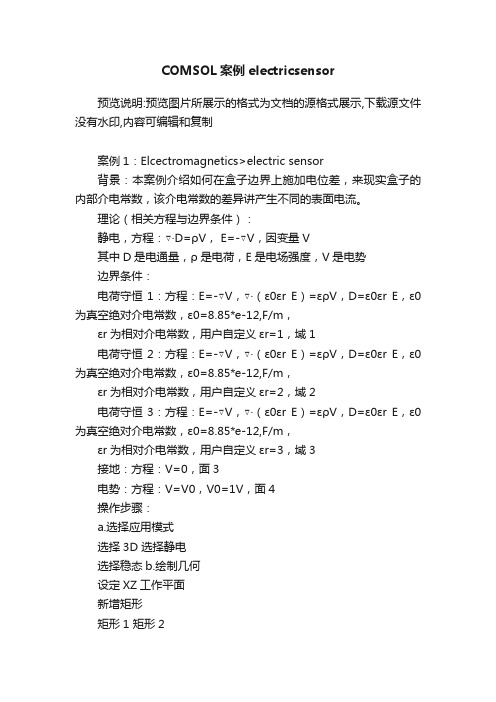
COMSOL案例electricsensor预览说明:预览图片所展示的格式为文档的源格式展示,下载源文件没有水印,内容可编辑和复制案例1:Elcectromagnetics>electric sensor背景:本案例介绍如何在盒子边界上施加电位差,来现实盒子的内部介电常数,该介电常数的差异讲产生不同的表面电流。
理论(相关方程与边界条件):静电,方程:▽·D=ρV, E=-▽V,因变量V其中D是电通量,ρ是电荷,E是电场强度,V是电势边界条件:电荷守恒1:方程:E=-▽V,▽·(ε0εr E)=ερV,D=ε0εr E,ε0为真空绝对介电常数,ε0=8.85*e-12,F/m,εr为相对介电常数,用户自定义εr=1,域1电荷守恒2:方程:E=-▽V,▽·(ε0εr E)=ερV,D=ε0εr E,ε0为真空绝对介电常数,ε0=8.85*e-12,F/m,εr为相对介电常数,用户自定义εr=2,域2电荷守恒3:方程:E=-▽V,▽·(ε0εr E)=ερV,D=ε0εr E,ε0为真空绝对介电常数,ε0=8.85*e-12,F/m,εr为相对介电常数,用户自定义εr=3,域3接地:方程:V=0,面3电势:方程:V=V0,V0=1V,面4操作步骤:a.选择应用模式选择3D 选择静电选择稳态b.绘制几何设定XZ工作平面新增矩形矩形1 矩形2矩形3并集运算图形显示新增椭圆椭圆1椭圆2编写制定运算图形显示拉伸设定新增长方体参数设定图形显示c.边界条件电荷守恒1电荷守恒2电荷守恒3接地设定电势设定d.网格剖分单元尺寸设定e.计算,求解。
comsol仿真案例
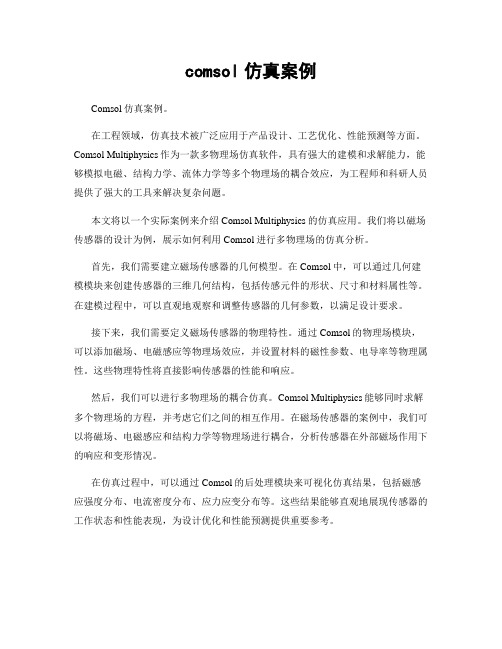
comsol仿真案例Comsol仿真案例。
在工程领域,仿真技术被广泛应用于产品设计、工艺优化、性能预测等方面。
Comsol Multiphysics作为一款多物理场仿真软件,具有强大的建模和求解能力,能够模拟电磁、结构力学、流体力学等多个物理场的耦合效应,为工程师和科研人员提供了强大的工具来解决复杂问题。
本文将以一个实际案例来介绍Comsol Multiphysics的仿真应用。
我们将以磁场传感器的设计为例,展示如何利用Comsol进行多物理场的仿真分析。
首先,我们需要建立磁场传感器的几何模型。
在Comsol中,可以通过几何建模模块来创建传感器的三维几何结构,包括传感元件的形状、尺寸和材料属性等。
在建模过程中,可以直观地观察和调整传感器的几何参数,以满足设计要求。
接下来,我们需要定义磁场传感器的物理特性。
通过Comsol的物理场模块,可以添加磁场、电磁感应等物理场效应,并设置材料的磁性参数、电导率等物理属性。
这些物理特性将直接影响传感器的性能和响应。
然后,我们可以进行多物理场的耦合仿真。
Comsol Multiphysics能够同时求解多个物理场的方程,并考虑它们之间的相互作用。
在磁场传感器的案例中,我们可以将磁场、电磁感应和结构力学等物理场进行耦合,分析传感器在外部磁场作用下的响应和变形情况。
在仿真过程中,可以通过Comsol的后处理模块来可视化仿真结果,包括磁感应强度分布、电流密度分布、应力应变分布等。
这些结果能够直观地展现传感器的工作状态和性能表现,为设计优化和性能预测提供重要参考。
最后,我们可以通过参数化设计和优化算法,对传感器的关键参数进行调整和优化。
Comsol Multiphysics提供了丰富的参数化建模和优化工具,能够快速高效地进行设计方案的评估和优化,以实现传感器性能的最大化。
总的来说,Comsol Multiphysics作为一款多物理场仿真软件,能够为工程师和科研人员提供强大的仿真分析工具,帮助他们解决复杂的工程和科学问题。
Comsol经典实例024:平行载流导线上的电磁力

在COMSOL Multiphysics 5.5版本中创建Comsol 经典实例024:平行载流导线上的电磁力此模型介绍通有恒定电流的两根平行导线的装置。
导线的横截面持续减小,直至达到设置的单位长度的力。
一、案例简介在无限长且可忽略圆形横截面的两根平行直导线中施加 1 安培的恒定电流,两根导线 相隔1米放在真空中,每米长度会产生2×10-7牛顿力 (N/m)。
本模型介绍遵循这个定义描述的两根平行导线的设置,但不同的是,导线存在有限横截面。
对于此示例中带有均匀电流密度的圆形横截面导线,相互作用的磁力与线电流相同。
这可以通过以下论据来理解:首先是两根导线都是线电流 (I) 的情况。
每个线电流都受洛伦兹力 (I×B) 支配,其中磁通密度 (B) 是由另一根导线产生的。
现在,为一根导线指定一个有限半径。
可直接从圆对称和麦克斯韦 - 安培定律得出,在这根导线外部, 产生的通量密度与之前完全相同,因此,其余线电流上的力是不变的。
而且,带分布 式电流密度的导线上的净力必须与线电流上的力大小完全相同 (但方向相反),这样, 力也不会改变。
如果两根导线交换位置,力必须仍然相同,由对称可推断出,只要导 线横截面不相交,则力与导线半径无关。
导线甚至可以是圆柱壳体或具有圆对称的任 何其他形状。
对于实验装置,需要横截面足够小,因为由导线和霍尔效应产生的阻抗 压降可能会导致产生随导线半径增大而增大的静电力,但此示例中没有考虑这种效应。
导线之间的力通过两种不同方法来计算:首先自动积分边界上的应力张量,然后积分导线横截面上的体积 (洛伦兹)力密度。
结果收敛于1A 定义的2×10-7N/m ,与预期相同。
二、模型定义本 App 通过 “二维磁场”接口构建。
建模平面是两根导线及其周围空气的横截面。
此方程式假设磁矢势的唯一非零分量为 A z ,这对应于与建模平面垂直的所有电流。
求解以下方程:e ()Z Z A J μ∇⨯⨯=1T2是空气的应力张量。
COMSOL案例详解-单相扩散 中文models.mfl.controlled_diffusion_micromixer_CN

2 在 ' 几何 ' 的 ' 设定 ' 窗口中找到 ' 单位 ' 栏,在 ' 长度单位 ' 列表中选择 'μm'。
可以通过拉伸二维工作平面来绘制几何。首先在工作平面中绘制结构的俯视 图。 3 在 ' 几何 ' 工具条中点击 ' 工作平面 ' 。
出口 1
增加含压力边界条件的出口。 1 在 ' 物理场 ' 工具条中点击 ' 边界 ' 并选择 ' 出口 '。 2 只选择边界 20 和边界 22。
此示例中缺省压力为 0 Pa。
对称 1
在对称平面中增加对称边界条件。 1 在 ' 物理场 ' 工具条中点击 ' 边界 ' 并选择 ' 对称 '。 2 只选择边界 4。
矩形 2
1 右键 ' 平面几何 ' 并选择 ' 矩形 '。 2 在矩形的 ' 设定 ' 窗口中找到 ' 尺寸与形状 ' 栏。
- 在 ' 宽度 ' 编辑框中输入:120。 - 在 ' 高度 ' 编辑框中输入:50。 3 找到 ' 位置 ' 栏。 - 在 'xw' 编辑框中输入:10。 - 在 'yw' 编辑框中输入:10。 4 点击 ' 构建选定 ' 按钮 。
。 4 点击 ' 增加 '。 5 在 ' 选择物理场 ' 下拉菜单中的 ' 化学物质传递 ' 下点击 ' 稀物质传递(tds)' 。 6 点击 ' 增加 ',然后点击 ' 研究 ' 按钮 。 7 在 ' 预置研究 ' 下拉菜单中点击 ' 稳态 ' 。 8 点击 ' 完成 ' 。
- 1、下载文档前请自行甄别文档内容的完整性,平台不提供额外的编辑、内容补充、找答案等附加服务。
- 2、"仅部分预览"的文档,不可在线预览部分如存在完整性等问题,可反馈申请退款(可完整预览的文档不适用该条件!)。
- 3、如文档侵犯您的权益,请联系客服反馈,我们会尽快为您处理(人工客服工作时间:9:00-18:30)。
info@
声源
• 入射波辐射条件 –开边界问题 • 法向加速度 –已知结构振动 • 驱动力 –未知结构振动 • 背景声压 –远处声源
中仿科技---专业信息化软件及技术咨询公司 CnTech Co.,Ltd--- Leading Engineering Virtual Prototyping Solutions Provider
info@
声源
• 入射波辐射条件 –开边界问题 • 法向加速度 –已知结构振动 • 驱动力 –未知结构振动 • 背景声压 –远处声源
中仿科技---专业信息化软件及技术咨询公司 CnTech Co.,Ltd--- Leading Engineering Virtual Prototyping Solutions Provider
实例:瞬态高斯脉冲波 COMSOL 4.1
中仿科技---专业信息化软件及技术咨询公司 CnTech Co.,Ltd--- Leading Engineering Virtual Prototyping Solutions Provider
info@
模型定义
• 本案例模拟瞬态高斯脉冲。椭圆为硬声场边界,左焦点发 出的声波会在 b/c秒后再次聚焦于右焦点,b为椭圆长轴的 长度,c为声速。声压方程为:
• 左焦点为高斯脉冲波,一波长剖分6单元 • 当t=1/f0时,波形如右图
气流速度
中仿科技---专业信息化软件及技术咨询公司 CnTech Co.,Ltd--- Leading Engineering Virtual Prototyping Solutions Provider info@
中仿科技---专业信息化软件及技术咨询公司 CnTech Co.,Ltd--- Leading Engineering Virtual Prototyping Solutions Provider
info@
模型简介
• 本案例模拟浸没在水中的中空圆柱体(内部充满水)受到 点声源或线声源激发时的声场分布(f=60kHz) • 3D模型: • 1.声波与结构双向耦合 • 2.分别模拟线声源和点声源
• 求解声压,p
–1 atm = 100 kPa –p ~ 1 – 100 Pa
• 频域中的控制方程
p 0
2 p 0 c2 0
info@
中仿科技---专业信息化软件及技术咨询公司 CnTech Co.,Ltd--- Leading Engineering Virtual Prototyping Solutions Provider
演示模型:房间的特征模式 COMSOL 4.1
中仿科技---专业信息化软件及技术咨询公司 CnTech Co.,Ltd--- Leading Engineering Virtual Prototyping Solutions Provider
info@
info@
声源
• 入射波辐射条件 –开边界问题 • 法向加速度 –已知结构振动 • 驱动力 –未知结构振动 • 背景声压 –远处声源
中仿科技---专业信息化软件及技术咨询公司 CnTech Co.,Ltd--- Leading Engineering Virtual Prototyping Solutions Provider
求解域声压传输方程
外层辐射边界条件 (最大限度减少声波反射的影响) 边界条件 交界水面 圆柱对声波
中仿科技---专业信息化软件及技术咨询公司 CnTech Co.,Ltd--- Leading Engineering Virtual Prototyping Solutions Provider info@
中仿科技---专业信息化软件及技术咨询公司 CnTech Co.,Ltd--- Leading Engineering Virtual Prototyping Solutions Provider info@
实例:中空圆柱体的声固耦合 COMSOL 4.1
声波对圆柱
结果与讨论
线声源辐射时声压级切面图 和圆柱体变形图
点声源辐射时声压级切面图 和圆柱体变形图
中仿科技---专业信息化软件及技术咨询公司 CnTech Co.,Ltd--- Leading Engineering Virtual Prototyping Solutions Provider
info@
模型定义,续
• f0与频宽成正比,f0=c/(Nh),h为网格单元尺寸,N为每个 波长内剖分的网格数。本模型取N=6.当t=1/f0时,波形和 完整的高斯波非常近似。 • 高斯函数的傅里叶变换非常简单(忽略截止效应):
中仿科技---专业信息化软件及技术咨询公司 CnTech Co.,Ltd--- Leading Engineering Virtual Prototyping Solutions Provider info@
压力声学
• 应用于流体
–空气和水最常见 –人体组织 (70% 水) –布、玻璃丝、石纤维
中仿科技---专业信息化软件及技术咨询公司 CnTech Co.,Ltd--- Leading Engineering Virtual Prototyping Solutions Provider
info@
模型介绍
• 本模型的房间尺寸为542.6m,里面有一台 电视机、两个喇叭以及一个长沙发。用声压分 布图直观地说明音乐的影响,计算所有低于 100Hz的特征频率及特征模式(eigenmode)。 • 特征模式显示了与之相 应的特征频率下声音的 强度模式。经由特征模 式的特性,可以得出结 论,应该将喇叭放置于 何处。
线声源辐射方程
点声源辐射方程
中仿科技---专业信息化软件及技术咨询公司 CnTech Co.,Ltd--- Leading Engineering Virtual Prototyping Solutions Provider
info@
模型定义
房间声场的特征模式
• 日常生活中共振有时会成为一个问题。客厅中的音乐 或家庭影院系统的低音能够摇动窗户及使地板震动。 这些现象发生于一些特定的频率——房间的特征频率。 • 音乐的体验会受到房间声场的特征模式影响。因此, 当设计一间音乐厅时,就必须考虑共振这一重要因素。 • 为了得到清澈且不受渲染的声音,特征频率应当均匀 地扩散。对于家庭影院或音乐系统的拥有者,由于无 法改变房间的外形,所以探讨喇叭应放置于何处能得 到较佳的声音是比较恰当的方法。
–与绝对压力无关 –仅为感兴趣的分布和特征频率
中仿科技---专业信息化软件及技术咨询公司 CnTech Co.,Ltd--- Leading Engineering Virtual Prototyping Solutions Provider
info@
COMSOL实例分析
中仿科技技术部
中仿科技---专业信息化软件及技术咨询公司 CnTech Co.,Ltd--- Leading Engineering Virtual Prototyping Solutions Provider
info@
声学模块的典型应用领域
喇叭
消声器
汽车回音
航空发动机
声固耦合
声压传感器
info@
中仿科技---专业信息化软件及技术咨询公司 CnTech Co.,Ltd--- Leading Engineering Virtual Prototyping Solutions Provider
中仿科技---专业信息化软件及技术咨询公司 CnTech Co.,Ltd--- Leading Engineering Virtual Prototyping Solutions Provider info@
模型定义
• 本模型使用波动方程(wave equation)描述声音在空气 中的传播情形: • 其中p表示压力,c是声音的速度。假如空气被简谐振动 的来源引起运动(例如喇叭),而仅仅只有一种频率可 在房间中存在。由此原因借由下式找寻时间谐波(timeharmonic)是有意义的。 • 波动方程此时以声音扰动的振幅p简 化为Helmholtz方程: • 本模型假设所有边界——墙、地板、 天花板以及家具都是完美刚体(硬声 场边界),相当于法向速率为0。
info@
外加声源
• 湍流
–K-eps使涡流变得模糊不清 => 不适合 –气动声学方程需要势流 –低Re模型使用LES或可能的偶极子源
• 白(粉、黑、机械…)噪声
–模拟为(可能随机)振幅vs.频率
• 点源、单极子、偶极子、线源…
–没问题
info@
声源
• 入射波辐射条件 –开边界问题 • 法向加速度 –已知结构振动 • 驱动力 –未知结构振动 • 背景声压 –远处声源
•Fe
中仿科技---专业信息化软件及技术咨询公司 CnTech Co.,Ltd--- Leading Engineering Virtual Prototyping Solutions Provider
中仿科技---专业信息化软件及技术咨询公司 CnTech Co.,Ltd--- Leading Engineering Virtual Prototyping Solutions Provider info@
无源 – 特征频率分析
• 无声源
声学模块
• 全面处理流体和固体中的声波问题 • 支持时谐、瞬态、特征频率、以及模态等分析 • 特点
– 易用的振动分析 – 支持衰减材料、压电材料 – 完美匹配层概念(PML) – 远场后处理功能 – 气动声学
音箱声压级分布
微穿孔版消声器
中仿科技---专业信息化软件及技术咨询公司 CnTech Co.,Ltd--- Leading Engineering Virtual Prototyping Solutions Provider info@