烷基表面活性剂的性能-main
表面活性剂的分类及应用性能
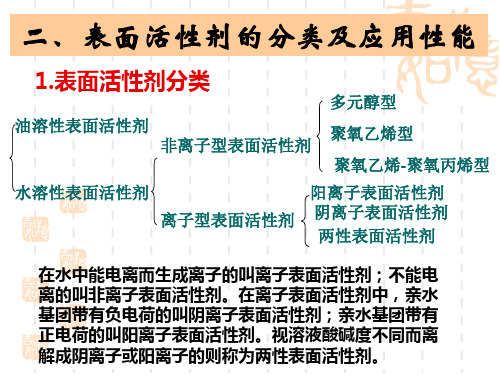
非离子型
高级醇聚氧 · · CH2Fra bibliotekH2 –(CH2CH2O)nH 乙烯加成物 CH3CH2·
发泡剂、乳 化剂、增溶 剂
按用量和品种,用的最多的是阴离子表面活性剂, 其次是非离子表面活性剂。阳离子表面活性剂,由 于它在纤维上的吸附大、洗涤力小,且价格昂贵, 不适合用于洗涤剂,有时在洗涤剂中加入阳离子表 面活性剂主要是为了使洗涤剂具有杀菌消毒能力或 起柔软作用。两性表面活性剂有良好的去污性能, 调理性好。但由于成本高而较少使用。常用于个人 卫生用品和特种洗涤剂中。因此,性能与成本的比 值是选择表面活性剂的一个主要依据。
2.表面活性剂的应用性能
表面活性剂因能对两相界面性质 产生影响,在实际应用中能显示 出各种优异的性能。在洗涤剂中, 表面活性剂一般作为洗涤成分, 但在某些配方中也用作辅助原料, 起乳化、润湿、增溶、保湿、润 滑、杀菌、柔软、抗静电、发泡、 消泡等作用。
表面活性剂在溶液中的性质
界面吸附
表面活性剂分子在界面上会定向排列成分 子层。如图所示:
表面活性剂的界面定向
表面活性剂在水溶液表面的吸附
表面活性剂在界面定向形成吸附膜
浓度足够时,表面活性剂在溶液表面定向形 成吸附膜。排列成单分子层。非极性憎水基的部 分越大,憎水性越强,表面活性剂分子就越聚集 于表面,其表面活性就越强。
形成胶束或胶团(micelle)
双亲分子溶解在水中达一定浓度时,其非极性部分会
◆ 增溶作用:表面活性剂在水溶液中达到CMC值 后,一些水不溶性或微溶性物质在胶束溶液中的 溶解度可显著增加,形成透明胶体溶液,这种作 用称为增溶(solubilization)。能产生增溶作用的表 面活性剂叫做增溶剂。增溶与胶束有关。由于胶 束的存在而使难溶物溶解度增加的现象统称为增 溶现象。 例如室温下苯在水中的溶解度很小,每100g水 只能溶解0.07g苯,但在10%的油酸钠水溶液中, 苯的溶解度达到7g/100g,增加了100倍,这是 通过油酸钠胶束的增溶作用实现的。 在药剂中,一些挥发油、脂溶性维生素、体激 素等许多难溶性药物常可借此增溶,形成澄明 溶液或提高浓度。
磺酸盐型芳烷基双子表面活性剂的合成及性能
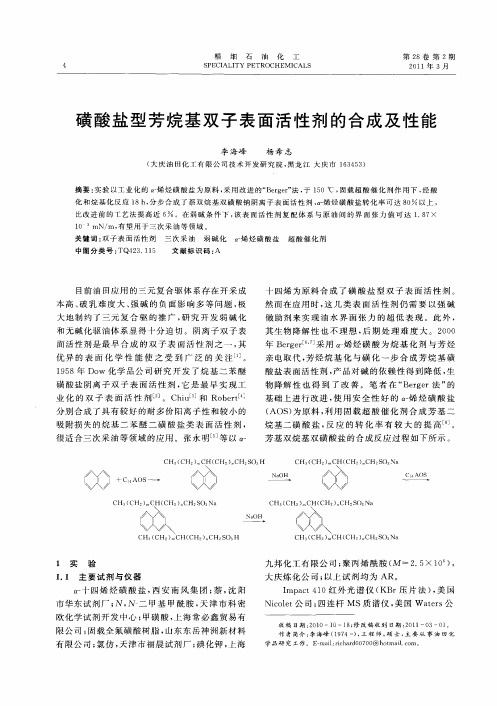
4
细
石
油
化
工
S PECI LI Y A T PET R0 CH EM I ALS C
第 2 8卷 第 2期 21 O 1年 3月
磺 酸 盐 型 芳 烷 基 双 子 表 面 活 性 剂 的 合 成 及 性 能
李 海 峰 杨 希 志
( 庆 油 田化 工 有 限公 司技 术 开 发 研 究 院 , 龙 江 大 庆 市 1 3 5 ) 大 黑 6 4 3
CH ( a CH ) CH ( 2 CH ) 2 CH S Na 2 O3
Na OH
CH CH2 CH ( 3( ) CH 2 CH ) Na ) 2 3 S(
CH ( 3 CH 2 CH ( ) CH ) 2 CH S 2 O3 H
CH3 CH ) ( 2 CH( CH 2 CH2 ) SO3 Na
摘 要 : 验 以 工 业 化 的 a 烯烃 磺 酸 盐 为 原 料 , 实 一 采用 改 进 的“ eg r法 , 1 O℃ , B r e” 于 5 固载 超 酸 催 化 剂 作 用 下 , 酸 经 化 和 烷 基 化 反 应 1 , 步 合 成 了 萘双 烷 基 双 磺 酸 钠 阴 离 子 表 面 活性 剂 ,一 8h 分 a 烯烃 磺 酸 盐 转 化 率 可 达 8 以上 , O 比改进 前 的 工艺 法 提 高 近 6 。在 弱 碱 条 件 下 , 表 面 活 性 剂 复 配 体 系 与 原 油 间 的 界 面 张 力 值 可 达 1 8 × 该 .7
1 。mN/ 有 望 用 于 三 次 采 油 等 领域 。 0 m,
关 键词 : 子 表 面 活性 剂 三 次 采 油 弱 碱 化 双
中 图 分 类号 : TQ4 3 1 5 2 . 1 文 献标 识 码 : A
十二烷基硫酸钠表面活性剂的制备及性能研究

十二烷基硫酸钠表面活性剂的制备及性能研究实验七十二烷基硫酸钠表面活性剂的制备及性能研究一、实验目的1、了解表面活性剂的基本性质及应用2、学习表面活性剂的分离纯化技术、学习表面活性剂性质的测试方法 3二、实验背景十二烷基硫酸钠, 别名为月桂醇硫酸钠, 是阴离子硫酸酯类表面活性剂的典型代表, 由于它具有良好的乳化性、起泡性、可生物降解、耐碱及耐硬水等特点, 广泛应用于化工、纺织、印染、制药、造纸、石油、化妆品和洗涤用品制造等各种工业部门。
表面活性剂的开发与应用已成为一个非常重要的行业,通过本综合实验让学生掌握表面活性剂研究的最基本实验技术和知识。
三、仪器和药品仪器:三口烧瓶,搅拌装置,分液漏斗,旋转蒸发器,抽滤装置,容量瓶(50mL),红外光谱分析仪,核磁共振,表面张力测定仪。
药品:正十二醇,氨基磺酸,尿素,浓硫酸,无水乙醇,氢氧化钠,乙醚,氯化钠,重蒸馏水。
四、实验提示1、查阅文献资料,依据实验室提供的条件,设计制备十二烷基硫酸钠的实验方案。
2、提出产物鉴定方法。
3、提出测定表面活性剂表面张力、临界胶束浓度的方法。
4、写出研究报告。
五、思考题1、采用氨基磺酸进行磺化反应的优点是什么,2、盐的加入对表面张力及临界胶束浓度有什么影响,六、参考文献[1]、陈敏,崔庆飞,氨基磺酸法合成十二烷基硫酸钠综合实验. 实验技术与管理,2007, 24(4): 35-37.[2]、陈联群, 李春兰, 叶莲, 等. 十二烷基硫酸钠的提纯与纯度测定. 内江师范学院学报, 2005, 20(6): 35-37.[3]、Alissa J. Prosser, Elias I. Franses, Newthermodynamic/electrostatic models of adsorption andtension equilibria of aqueous ionic surfactant mixtures: application to sodium dodecylsulfate/sodium dodecyl sulfonate systems, Journal of Colloid and Interface Science, 2003, 263:606-615The synthesize and properties study of sodium dodecyl sulfateThe experiment purposes1、Understanding the basic properties and application of surfactant2、Study the technology of isolation and purification for surfactant3、Study the measuremenst of surfactant propertiesBackgroundSodium dodecyl sulfate, SDS, other names for laurel sulfate, is a common type of anionic surfactant. It has excellent emulsifying, foaming property, biodegradability, good alkaline resistance, and hard water instance, etc. SDS is widely used in non-phosphorus washing powder,liquid detergents and home washing products, textile, printing and dyeing industry, petrochemical products, and industrial hard surface cleaning agents, etc. SDS is widely used in many fields such as chemical industry, textile, dyeing, pharmaceutical, paper making, oil field, detergent, cosmetic, personal hygiene aid industries and fields. Developing and application of surfactant has become an important field. The purpose of the comprehensive experiment is to Understanding the basic experiment technology and knowledge.Instruments and reagentsInstruments and apparatus: three-necked flask, agitator, pressure funnel,separalory funnel, rotaryevaporator, filtration apparatus, volumetric flask (50mL), FT-IR spectrometer, NMR equipment, surface tension meter.Reagents: dodecanol, aminosulfonic acid, urea, sulfuric acid, anhydrous alcohol, sodium hydroxide, ethyl ether, Sodium chloride, distilled water.The notice of experiment1、Consulting literature material and based on the Conditionprovided by laboratory, design the scheme of preparing SDS2、Proposes appraisal method of the product3、Proposes measurement methods for surface tension, criticalmicelle concentration 4、Provide the thesis.Question1、What is the advantages of the sulfonation reaction used aminosulfonic acid as sulfonation reagent?2、What is the effects of salt on surface tension and critical micelle concentration?Reference[1]、Chen M. Cui Q. F. The syntheses experiment of synthesis of sodium dodecyl sulfate from aminosulfonic acid, Experimental Technology and Management, 2007, 24(4): 35-37. [2]、Chen L. Q. Li C. L. Ye L. Zeng P. Zhu Y. P. Purification and purity determination of sodium dodecyl sulfate, Journal of Neijiang Teachers College, 2005, 20(6): 35-37.[3]、Alissa J. Prosser, Elias I. Franses, Newthermodynamic/electrostatic models of adsorption and tension equilibria of aqueous ionic surfactant mixtures: application to sodium dodecyl sulfate/sodium dodecyl sulfonate systems, Journal of Colloid and Interface Science, 2003, 263: 606-615合成步骤1、向配有搅拌装置的三口烧瓶中加入含40 mL正十二醇(过量部分起稀释作用),混合均匀的9.7g氨基磺酸和2.4g尿素,再滴加少量浓硫酸,在105~110 oC 下搅拌反应70 min,用30%的NaOH溶液处理,放尽NH3后倒出即得到粗产品。
表面活性剂烷基多苷
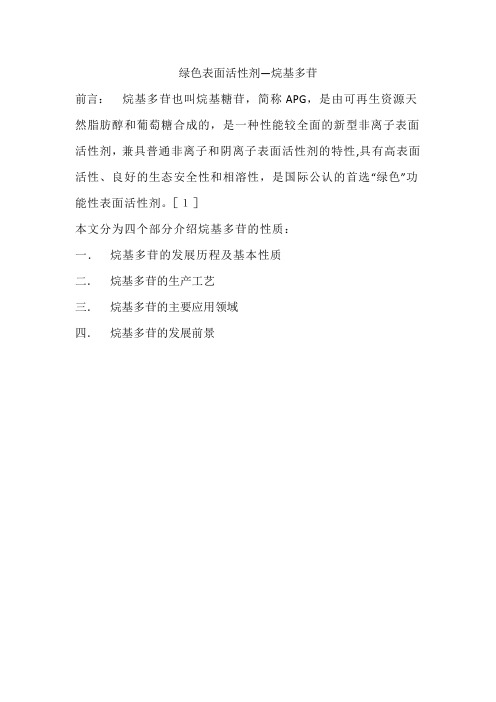
绿色表面活性剂—烷基多苷前言:烷基多苷也叫烷基糖苷,简称APG,是由可再生资源天然脂肪醇和葡萄糖合成的,是一种性能较全面的新型非离子表面活性剂,兼具普通非离子和阴离子表面活性剂的特性,具有高表面活性、良好的生态安全性和相溶性,是国际公认的首选“绿色”功能性表面活性剂。
[1]本文分为四个部分介绍烷基多苷的性质:一.烷基多苷的发展历程及基本性质二.烷基多苷的生产工艺三.烷基多苷的主要应用领域四.烷基多苷的发展前景绿色表面活性剂——烷基多苷学院:材料与化工学院班级:08级理科实验班学号:20080W0103 姓名:赵慧超Investigation on the Development andApplication of Green Surfactant Agent Abstract:Alkyl Polyglysoside (APG) is a new type of mild nonionic green surfactant with wide applications and excellent properties. The preparati -on , properties and application of APG are introduced in this paper .关键字:烷基多苷,绿色,可降解,无毒一.烷基多苷的发展历程及基本性质烷基多苷简称APG,系非离子表面活性剂, 它是由可再生资源淀粉的衍生物葡萄糖和脂肪醇为原料,由半缩醛羟基与醇羟基在酸催化剂存在下脱去一分子水得到的一种苷化合物。
[2]APG的特殊结构使它具有比其他乙氧基非离子表面活性剂更优异的性能,表现在无浊点,水稀释后无凝胶现象;APG兼有非离子表面活性剂和阴离子表面活性剂的特性。
[3]APG的研制已有100多年的历史,1978年法国的Seppic公司建成第一套(1500t/a)APG工业装置,才开始了APG的工业化生产。
表面活性剂洗涤剂的成分及性能
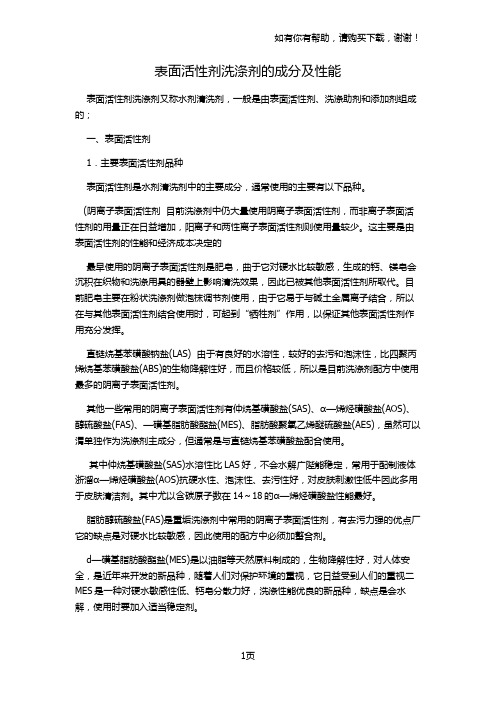
表面活性剂洗涤剂的成分及性能表面活性剂洗涤剂又称水剂清洗剂,一般是由表面活性剂、洗涤助剂和添加剂组成的;一、表面活性剂1.主要表面活性剂品种表面活性剂是水剂清洗剂中的主要成分,通常使用的主要有以下品种。
(阴离子表面活性剂目前洗涤剂中仍大量使用阴离子表面活性剂,而非离子表面活性剂的用量正在日益增加,阳离子和两性离子表面活性剂则使用量较少。
这主要是由表面活性剂的性能和经济成本决定的最早使用的阴离子表面活性剂是肥皂,曲于它对硬水比较敏感,生成的钙、镁皂会沉积在织物和洗涤用具的器壁上影响清洗效果,因此已被其他表面活性剂所取代。
目前肥皂主要在粉状洗涤剂做泡抹调节剂使用,由于它易于与碱土金属离子结合,所以在与其他表面活性剂结合使用时,可起到“牺牲剂”作用,以保证其他表面活性剂作用充分发挥。
直链烷基苯磺酸钠盐(LAS) 由于有良好的水溶性,较好的去污和泡沫性,比四聚丙烯烷基苯磺酸盐(ABS)的生物降解性好,而且价格较低,所以是目前洗涤剂配方中使用最多的阴离子表面活性剂。
其他一些常用的阴离子表面活性剂有仲烷基磺酸盐(SAS)、α—烯烃磺酸盐(AOS)、醇硫酸盐(FAS)、—磺基脂肪酸酯盐(MES)、脂肪酸聚氧乙烯醚硫酸盐(AES),虽然可以渭单独作为洗涤剂主成分,但通常是与直链烷基苯磺酸盐配合使用。
其中仲烷基磺酸盐(SAS)水溶性比LAS好,不会水解广陛能稳定,常用于配制液体浙溜α—烯烃磺酸盐(AOS)抗硬水性、泡沫性、去污性好,对皮肤刺激性低牛因此多用于皮肤清洁剂。
其中尤以含碳原子数在14~18的α—烯烃磺酸盐性能最好。
脂肪醇硫酸盐(FAS)是重垢洗涤剂中常用的阴离子表面活性剂,有去污力强的优点厂它的缺点是对硬水比较敏感,因此使用的配方中必须加螯合剂。
d—磺基脂肪酸酯盐(MES)是以油脂等天然原料制成的,生物降解性好,对人体安全,是近年来开发的新品种,随着人们对保护环境的重视,它日益受到人们的重视二MES是一种对硬水敏感性低、钙皂分散力好,洗涤性能优良的新品种,缺点是会水解,使用时要加入适当稳定剂。
表面活性剂的性能和作用
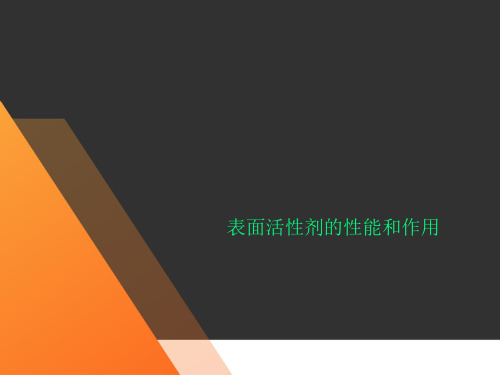
docin/sundae_meng
影响临界胶束浓度(CMC)的因素
• 2.支链的表面活性剂的cmc高于具有相同碳数的直链 的.
docin/sundae_meng
油 微乳状
H2O
docin/sundae_meng
临界胶束浓度
• 若在层状胶束中加入适量的非极性溶液,则 可能形成微乳液,形成反相胶束。
• 对表面活性剂聚集体的研究方兴未艾,已成 为有序组合体超分子化学和分子层面上的化 学研究部分。表面活性剂分子聚集效应可以 产生增溶中心和某些化学反应的微环境,形 成胶束反应器。(如纳米材料)。
• 3.增加不饱和键时, cmc相应增高;一般来说,每增一 个双键, cmc增大3~4倍.
• 4.碳氢链中有极性时, cmc显著增高. • 5.有苯环cmc增高. • 6.含碳氟链的, cmc显著减小.(具有较高的表面活性. • 7.离子cmc较非离子大100倍. • 8.在表面活性剂中添加盐,使cmc下降.二价比一价降
(一)胶束的形成 临界腔束浓度CMC: 表面活性剂分子缔合形成胶束的最低浓度。 胶体粒子范围(1~100nm) 临界浓度通常在0.02%~0.5%左右。
docin/sundae_meng
临界胶束结构
docin/sundae_meng
胶束(micelle)
docin/sundae_meng
胶束(micelle)
表面活性剂既亲水又亲油的两亲性,使其具有一部分可溶 于水而另一部分易从水中逃离的双重性质。在水溶液中, 尽管水分子与疏水基团存在着相互作用,但水分子之间的 作用力要远大于它们之间的作用,而疏水基团则存在着相 互吸引、相互缔合而离开水相的趋势。在水溶液中疏水基 团相互吸引、缔合的作用称为疏水作用(效应)。
表面活性剂的性能与应用原理
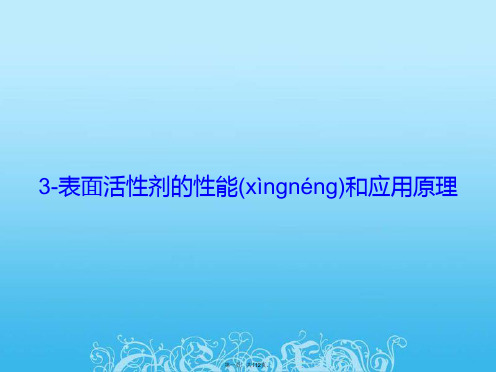
表面张力产生原因(yuányīn)、 定义
原因
液体内部分子受力平 衡
表面层分子受力不平 衡;合力指向液体内 部,表面层分子有被 拉进液体内部趋势 (qūshì)
定义
第十页,共112页。
表面张力(biǎomiànzhānglì) 定义
宏观表现为液体表面有收缩趋势(从而 处于表面层内分子数减少),或者说表 面恒有一种抵抗表面扩张的力,即表面 张力(σ,亦有用γ表示的)
表面(biǎomiàn)
界面:
相与相之间的接触面
固-液、固-固、固- 气、液-液、液-气
表面
两相中一相为气相时形 成的界面
固-气、液-气
说明(shuōmíng) 界面实际上并不是一个
几何面,它通常是约几 个分子厚度的过渡区
第六页,共112页。
表面张力 (biǎomiànz hānglì)
用途 是配制重垢液体洗涤剂的主要原料。可做个人卫生盥洗制品、各
种洗衣物以及硬表面清洗剂
使用时常与醇醚硫酸(AES),α—烯烃磺酸盐(AOS)复配,以弥补 SAS在硬水中泡沫性差的缺点
第二十八页,共112页。
α-烯烃(xītīng)磺酸盐
在硬水中和有肥皂存在时具有很好的起泡 力和优良的去污力;毒性低、对皮肤刺激 性小、性能(xìngnéng)温和
第十八页,共112页。
概述(ɡài shù)(常见分类方 法)
按SAA在水溶液中能否(nénɡ fǒu)解离及解离后所带电荷类型 非离子型、离子型(阴离子、阳离子和两性SAA) 按用途分类 如润湿剂、渗透剂、乳化剂、分散剂、柔软剂、抗静电剂、洗涤
剂等
按SAA在水和油中的溶解性 水溶性和油溶性SAA 按分子量分类 分子量大于10 000者称为高分子SAA;分子量在1000~10 000的
烷基苯磺酸盐表面活性剂的界面性能研究
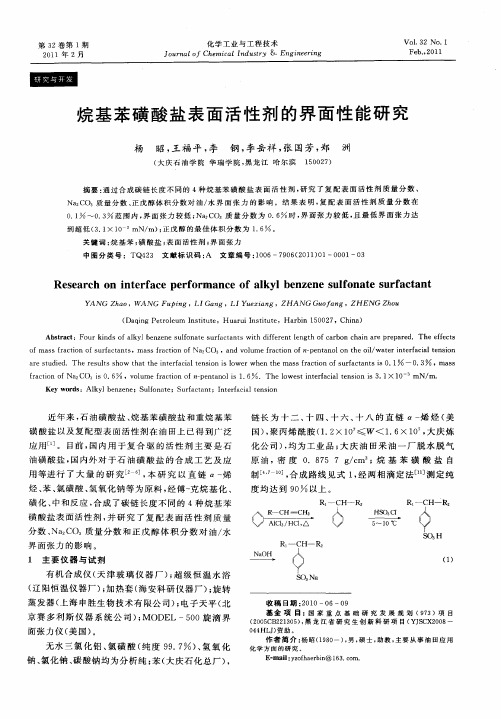
21 0 1年 2月
化 学 工 业 与 工 程 技 术 Jo r a fCh mia n u ty & En ie rn u n l e c lI d sr o g n e ig
Vo. 2 NO I 3 .1 Fe , O1l b.2 面 性 能 研 究
fa t n o 2 s0 6 ,v lmefa t no — e tn li 1 6 . Th o s n erca e so s3 1× 1 ~ mN/ r ci fNaCO3i . o o u rci f p na o s . o elwe titra iltn ini . 0 m.
Re e r h o n e f c e f r a c f a k lb nz ne s lo t u f c a t s a c n i t r a e p r o m n e o l y e e u f na e s r a t n
Y ANG a Zh o,WANG Fu n pig,LIG n a g,LIY e a g,ZHANG o n u xin Gu 厅 g,ZHENG o Zh u
( qng Pe r e Da i tolum n tt t H ua uiI tt e, H a bi 50 27,Ch n I s iu e, r ns iut r n1 0 i a) Ab ta t sr c :Fo nd fa ky e z n u f na e s f t nt ih if r ntlng h ofc r n c i e pr p r d T h fe t urki s o l lb n e e s lo t uraca sw t d fe e e t a bo han ar e a e . eefcs
表面活性剂的概念及性能指标

表面活性剂的概念及性能指标1、表面活性剂简介表面活性剂具有两性分子结构:一端是亲水基团,简称亲水基,也称为疏油基或憎油基,能够使表面活性剂以单体的形式溶在水中。
亲水基团经常为极性基团,可为羧基(-COOH)、磺酸基(-SO3H)、氨基(-NH2)或胺基及其盐,羟基(-OH)、酰胺基、醚键(-O-)等也可作为极性亲水基团;另一端是疏水基团,简称亲油基,也称为疏水基或憎水基。
疏水基团通常为非极性烃链,如疏水的烷基链R-(烷基)、Ar-(芳基)等。
表面活性剂分为离子型表面活性剂(包括阳离子表面活性剂与阴离子表面活性剂)、非离子型表面活性剂、两性表面活性剂、复配表面活性剂、其他表面活性剂等。
表面活性剂溶液中,表面活性剂的浓度达到一定值后,表面活性剂分子会形成各种有序的组合体称之为胶束。
胶束化作用或胶束的形成是表面活性剂溶液十分重要的基本性质,一些重要的界面现象都与胶束的形成有关。
使表面活性剂在溶液中形成胶束的浓度被称之为临界胶束浓度(Critical Micelle Concentration,CMC)。
胶束并不是固定不变的球形,它为极度不规则、动态变化的形状。
在某些条件下,表面活性剂还会出现反胶束的状态。
影响临界胶束浓度主要因素:表面活性剂的结构、添加剂的加入与类型、温度的影响。
2、表面活性剂与蛋白质相互作用蛋白质包含非极性、极性和带电基团,许多两亲分子均可以与蛋白质发生各种作用。
表面活性剂在不同条件下可形成具有不同结构的分子有序组合体,如胶束、反胶束等,其分别与蛋白质相互作用也不同。
蛋白质-表面活性剂(Protein-Surfactant,P-S)之间主要存在着静电作用和疏水作用,离子型表面活性剂与蛋白质作用主要是极性基的静电作用和疏水碳氢链的疏水作用,分别结合到蛋白质的极性和疏水部分,形成P-S的复合物。
而非离子型表面活性剂主要通过疏水力与蛋白质发生作用,其疏水链与蛋白质的疏水基团之间的相互作用对表面活性剂和蛋白质的结构和功能都能产生一定的影响。
油烷基Gemini表面活性剂的流变性能研究
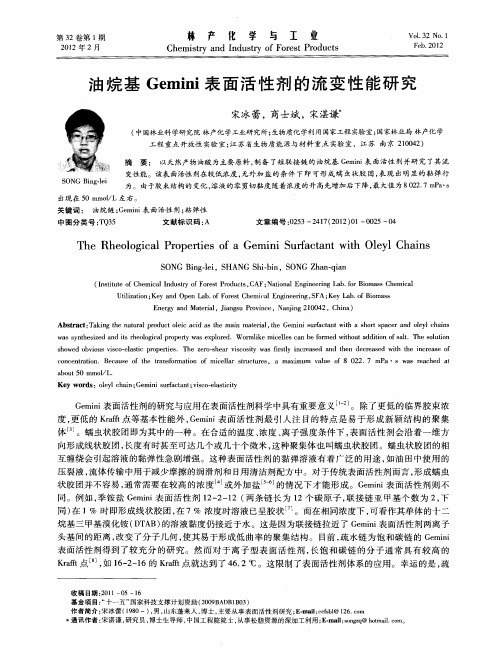
油 烷 基 Ge n 表 面 活 性 剂 的 流 变 性 能 研 究 mii
宋冰蕾 , 商士斌 , 宋湛
( 中国林业科学研究院 林产化 学工业研 究所 ; 生物质化学利用 国家工程 实验 室; 国家林业局 林产化学
工程 重 点 开 放 性 实验 室 ; 苏省 生 物 质 能 源与 材 料 重 点 实验 室 ,江 苏 南京 2 04 ) 江 10 2
向形成线状胶团 , 长度有时甚至可达几个或几十个微米 , 这种聚集体也叫蠕虫状胶 团。蠕虫状胶 团的相 互缠 绕 会 引起溶 液 的黏 弹性 急剧 增强 。这 种 表面 活性 剂 的黏 弹 溶 液有 着 广 泛 的用 途 , 油 田中使 用 的 如 压裂 液 , 流体 传 输 中用 于减少 摩擦 的润滑剂 和 日用 清 洁剂 配方 中 。对 于 传统表 面 活性剂 而 言 , 成蠕 虫 形
Ut ia in; y a d Op n L b o o e tC e c lE g n e i g S A ; y L b o o s i zt l o Ke n e a . fF r s h mia n i e r , F Ke a . fBima s n
E eg n tr l J ns rv c , aj g 0 2 hn ) n r adMa i , i guPoi e N ni 1 4 ,C ia y ea a n n 2 0
c n e r to . Be a s o he r n f r to o iel r tucu e o c ntai n c u e f t ta so ma in f m c la sr tr s, a a mum v l e f 8 2 m xi au o 0 2.7 m P ・S a wa rac d t s e he a a o 0 mm o/L. b ut5 1 K e o ds:o e lc a n; m i u fc a t v s o ea tct yw r ly h i Ge nis ra t n ; ic — lsiiy
表面活性剂的性能测定与评价
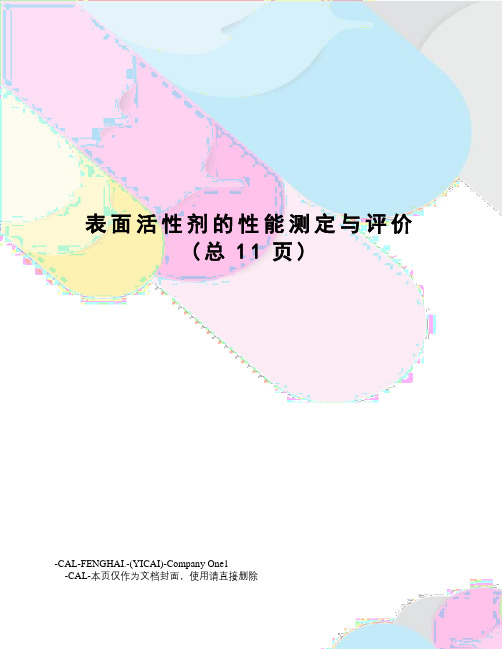
表面活性剂的性能测定与评价(总11页)-CAL-FENGHAI.-(YICAI)-Company One1-CAL-本页仅作为文档封面,使用请直接删除中国石油大学(油田化学基础实验)实验报告实验日期:成绩:班级:石工学号:1302姓名:教师:同组者:表面活性剂的性能测定及评价一.实验目的1、了解用指示剂和染料通过显色反应鉴别表面活性剂类型的原理和方法;2、了解离子型表面活性剂克拉夫特点和非离子表面活性剂浊点的测定方法及不同类型表面活性剂的性质;3、学会一种表面活性剂的界面张力的测定原理和方法,并掌握由表面张力计算临界胶束浓度(CMC)的原理和方法,学习Gibbs公式及其应用;4、学会表面活性剂溶液与原油的油水界面张力的测定原理和方法,并掌握超低界面张力在三次采油中的作用机理;5、学会观察表面活性剂溶液与原油混合后的乳化现象,并掌握不稳定体系数法评价表面活性剂的乳化能力。
二.实验原理表面活性剂分子是由亲水性的极性基团和憎水性的的非极性基团所组成的有机化合物,当它们一低浓度存在于某一体系中时,可被吸附在该体系的表面上,采取极性基团向着水,非极性基团脱离水的表面定向,从而使表面自由能明显降低。
1、表面活性剂类型的鉴别不同类型的表面活性剂具有不同的性质,因此可采用不同的方法将它们鉴别出来。
离子表面活性剂可利用他们的离子反应来鉴别,非离子表面活性剂则利用其与金属离子形成络合物的颜色来鉴别。
亚甲基蓝属阳离子型有色物,在容量分析中可作指示剂使用,当它遇阴离子表面活性剂时,生成不溶于水而溶于氯仿的产物,使氯仿层色泽变深;如果实验液中含有阳离子表面活性剂,由于阴阳离子表面活性剂的结合,使亚甲基蓝脱离阴离子表面活性剂而从氯仿中重新回到水中,使氯仿色泽变浅。
2、表面活性剂克拉夫特点和浊点离子型表面活性剂在温度较低时溶解度很小,但随温度升高而逐渐增加,当到达某特定温度时,溶解度急剧陡升,把该温度称为临界溶解温度又称克拉夫特点。
17种常用表面活性剂特性及使用方法简介

17种常用表面活性剂特性及使用方法简介月桂基磺化琥珀酸单酯二钠(DLS)一、英文名: Disodium Monolauryl Sulfosuccinate二、化学名:月桂基磺化琥珀酸单酯二钠三、化学结构式: ROCO-CH2-CH(SO3Na)-COONa四、产品特性1. 常温下为白色细腻膏体,加热后(>70℃)为透明液体;2. 泡沫细密丰富;无滑腻感,非常容易冲洗;3. 去污力强,脱脂力低,属常见的温和性表面活性剂;4. 能与其它表面活性剂配伍,并降低其刺激性;5. 耐硬水,生物降解性好,性能价格比高。
五、技术指标:1.外观(25℃):纯白色细腻膏状体2.含量(%): 48.0—50.03.Na2SO3(%):≤0.504.PH值(1%水溶液):5.5—7.0六、用途与用量:1.用途:配制温和高粘度高度清洁的洗手膏(液)、泡沫洁面膏、泡沫洁面乳、泡沫剃须膏,也可配制爽洁无滑腻的泡沫沐浴露、珠光香波等。
2.推荐用量:10—60%。
脂肪醇聚氧乙烯醚(3)磺基琥珀酸单酯二钠MES一、英文名:Disodium Laureth(3) Sulfosuccinate二、化学名:脂肪醇聚氧乙烯醚(3)磺基琥珀酸单酯二钠三、化学结构式:RO(CH2CH2O)3COCH2CH(SO3Na)COONa四、产品特性:2.刺激性低,且能显著降低其他表面活性剂的刺激性;3.泡沫丰富细密稳定;性能价格比高;4.有优良的钙皂分散和抗硬水性能;5.复配性能好,能与多种表面活性剂和植物提取液(如皂角、首乌)复配,形成十分稳定的体系,创制天然用品;6.脱脂力低,去污力适中,极易冲洗且无滑腻感。
五、技术指标:1.外观(25℃):无色至浅黄色透明粘稠液体2.活性物(%): 30.0±2.03.PH值(1%): 5.5—6.53.色泽(APHA):≤504.Na2SO3 (%):≤0.35.泡沫(mm):≥150六、用途与用量:1、用途:制造洗发香波、泡沫浴、沐浴露、洗手液、外科手术清洗及其它化妆品、洗涤日化产品等,还可作为乳化剂、分散剂、润湿剂、发泡剂等。
比较全的常用表面活性剂特性
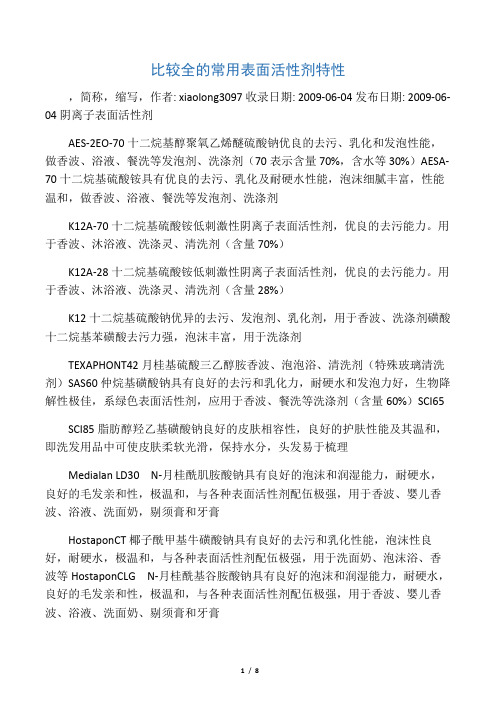
比较全的常用表面活性剂特性,简称,缩写,作者: xiaolong3097收录日期: 2009-06-04发布日期: 2009-06-04阴离子表面活性剂AES-2EO-70十二烷基醇聚氧乙烯醚硫酸钠优良的去污、乳化和发泡性能,做香波、浴液、餐洗等发泡剂、洗涤剂(70表示含量70%,含水等30%)AESA-70十二烷基硫酸铵具有优良的去污、乳化及耐硬水性能,泡沫细腻丰富,性能温和,做香波、浴液、餐洗等发泡剂、洗涤剂K12A-70十二烷基硫酸铵低刺激性阴离子表面活性剂,优良的去污能力。
用于香波、沐浴液、洗涤灵、清洗剂(含量70%)K12A-28十二烷基硫酸铵低刺激性阴离子表面活性剂,优良的去污能力。
用于香波、沐浴液、洗涤灵、清洗剂(含量28%)K12十二烷基硫酸钠优异的去污、发泡剂、乳化剂,用于香波、洗涤剂磺酸十二烷基苯磺酸去污力强,泡沫丰富,用于洗涤剂TEXAPHONT42月桂基硫酸三乙醇胺香波、泡泡浴、清洗剂(特殊玻璃清洗剂)SAS60仲烷基磺酸钠具有良好的去污和乳化力,耐硬水和发泡力好,生物降解性极佳,系绿色表面活性剂,应用于香波、餐洗等洗涤剂(含量60%)SCI65SCI85脂肪醇羟乙基磺酸钠良好的皮肤相容性,良好的护肤性能及其温和,即洗发用品中可使皮肤柔软光滑,保持水分,头发易于梳理Medialan LD30 N-月桂酰肌胺酸钠具有良好的泡沫和润湿能力,耐硬水,良好的毛发亲和性,极温和,与各种表面活性剂配伍极强,用于香波、婴儿香波、浴液、洗面奶,剔须膏和牙膏HostaponCT椰子酰甲基牛磺酸钠具有良好的去污和乳化性能,泡沫性良好,耐硬水,极温和,与各种表面活性剂配伍极强,用于洗面奶、泡沫浴、香波等HostaponCLG N-月桂酰基谷胺酸钠具有良好的泡沫和润湿能力,耐硬水,良好的毛发亲和性,极温和,与各种表面活性剂配伍极强,用于香波、婴儿香波、浴液、洗面奶、剔须膏和牙膏Ganapol AMG酰胺基聚氧乙烯醚硫酸镁用于婴儿和温和香波、沐浴制品、洗面奶和极温和清洁化妆品SandopanLS-24月桂醇聚氧乙烯醚羧酸钠具有良好的去污和乳化性能,泡沫性良好,耐硬水,极温和,与各种表面活性剂配伍极强,用于洗面奶、泡沫浴、香波等MAP-85十二烷基磷酸酯医用级,乳化,由于其溶解特性,需于KOH,铵盐中和,泡沫丰富而细腻MAP-K十二烷基磷酸酯钾盐优良的乳化、分散、洗涤、抗静电性,温和无刺激,配伍性好,对头发有明显润泽作用,用于洗面奶、香波、浴液中,泡沫稠密、稳定,洗后皮肤润泽MAP-A十二烷基磷酯酯三乙醇胺优良的乳化、分散、洗涤、抗静电性,温和无刺激,配伍性好,对头发有明显润泽作用,用于洗面奶、香波、浴液中,泡沫稠密、稳定,洗后皮肤润泽MES十二醇聚氧乙烯醚磺基琥珀酸酯二钠性能温和,有效降低其它表面活性剂的刺激性,泡沫丰富,有乳化分散、增溶能力,配伍性好,用于婴儿香波、洗面奶、浴液AOSα-烯基磺酸钠用于轻垢洗涤剂、洗手剂、香波、液体皂及油田助剂非离子表面活性剂COMPERLAN100C椰油脂肪酸单乙醇酰胺良好的增稠稳泡剂,用于香波、沐浴露、珠光浆、盥洗室用品等COMPERLANCOD椰油脂肪酸二乙醇酰胺良好的增稠稳泡剂,用于香波、沐浴露、珠光浆、盥洗室用品等GLUCOPON600CSUP(APG)C12-14烷基糖苷可生物降解。
常见固体表面活性剂性能及应用介绍
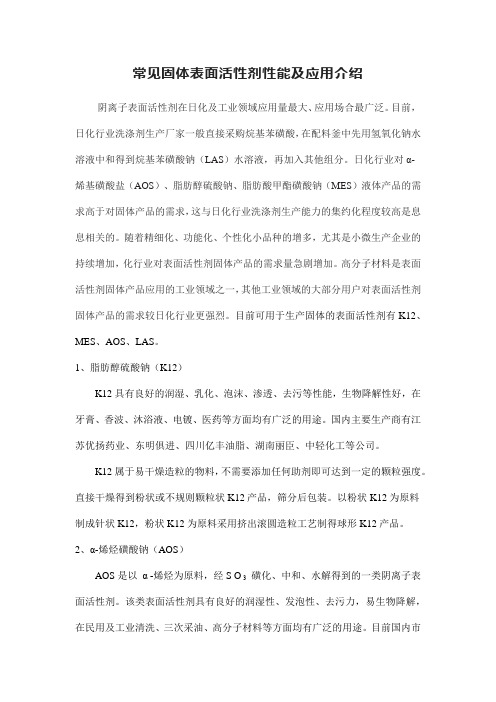
常见固体表面活性剂性能及应用介绍阴离子表面活性剂在日化及工业领域应用量最大、应用场合最广泛。
目前,日化行业洗涤剂生产厂家一般直接采购烷基苯磺酸,在配料釜中先用氢氧化钠水溶液中和得到烷基苯磺酸钠(LAS)水溶液,再加入其他组分。
日化行业对α-烯基磺酸盐(AOS)、脂肪醇硫酸钠、脂肪酸甲酯磺酸钠(MES)液体产品的需求高于对固体产品的需求,这与日化行业洗涤剂生产能力的集约化程度较高是息息相关的。
随着精细化、功能化、个性化小品种的增多,尤其是小微生产企业的持续增加,化行业对表面活性剂固体产品的需求量急剧增加。
高分子材料是表面活性剂固体产品应用的工业领域之一,其他工业领域的大部分用户对表面活性剂固体产品的需求较日化行业更强烈。
目前可用于生产固体的表面活性剂有K12、MES、AOS、LAS。
1、脂肪醇硫酸钠(K12)K12具有良好的润湿、乳化、泡沫、渗透、去污等性能,生物降解性好,在牙膏、香波、沐浴液、电镀、医药等方面均有广泛的用途。
国内主要生产商有江苏优扬药业、东明俱进、四川亿丰油脂、湖南丽臣、中轻化工等公司。
K12属于易干燥造粒的物料,不需要添加任何助剂即可达到一定的颗粒强度。
直接干燥得到粉状或不规则颗粒状K12产品,筛分后包装。
以粉状K12为原料制成针状K12,粉状K12为原料采用挤出滚圆造粒工艺制得球形K12产品。
2、α-烯烃磺酸钠(AOS)AOS是以α -烯烃为原料,经S O 3磺化、中和、水解得到的一类阴离子表面活性剂。
该类表面活性剂具有良好的润湿性、发泡性、去污力,易生物降解,在民用及工业清洗、三次采油、高分子材料等方面均有广泛的用途。
目前国内市场上AOS 碳链长度一般为C14~16或C14~18,主要有两种产品形式:35%含量左右的液体和90%含量以上的粉状。
不同碳数AOS 固体产品的状态不同,C14 和C 12~14 AOS的水分含量3%~4%时为松脆的固体,C 14~18 AOS 在水分含量低于5%时较为松散。
新型表面活性剂Gmini

4.相转移催化剂
1971年,Bunton等将Gemini阳离子表面活性剂(C16一4一C16,2Br‘和C16一4一C16,2Br- ) 应用于水解反应,发现该类表面活性剂的催化效率是其相应单体表面活性剂的2一5倍;并且所 需Gemini阳离子型表面活性剂的催化浓度更低。Bunton等还将C16一s一C16,ZBf应用于催化 6一硝基苯氧氮杂茂一3一羧酸盐脱梭反应。在研究正十二烯在水/有机溶剂中的氢甲酞化反 应时,Chen等I’“2]发现:与相应的单体表面活性剂相比(C16一s一C16,2Br-,s=2,4,6)更有效的提 高反应的选择性和反应速度。由于Gemini阳离子型表面活性剂具有较低的CMC,在较低的浓 度下就能够形成聚集体,对正十二烯的增溶能力更强,有利于反应物分子传输到两相反应界面, 从而更有效地降低相转移的自由能。Qiu等在研究2,4一二硝基氯代苯碱性条件下水解时发现, 在浓度较低时(<5mM), C12一4一C12,2Br与C12TAB的催化机制相似,但当表面活性剂的浓度 大于5mM时, C12一4一C12,2Br-的催化效率比其相应的单体表面活性剂高许多。Liu等以C16一 3一C16,2Br‘的水溶液为反应介质合成纳米材料一银丝,能够制备平均直径为30纳米、长度为 几到十几微米的高质量的银丝。
目前对Gemini非离子表面活性剂的研究报道相对较少,亲水基团主要为聚氧乙烯链和 糖基。
4.4Gemini两性离子表面活性剂和杂双子表面活性剂的合成
由于在合成方面存在一定难度,目前这两类Gemini表面活性剂的研究报道相对较
少,但这两类Gemini表面活性剂具有更独特的性能,也具有潜在的应用价值。
5.Gemini表面活性剂的应用
1、微乳燃料。
“微乳状液是一个由水、油和两亲物质(分子)组成的、光学上各相同性、 热力学上稳定的溶液体系”,在外观上呈透明和半透明状。1943 年,苏尔曼 (Schulman)和霍尔(Hoar)首次报道了这一分散体系。这种分散体系,可 以是油分散在水中(O/W型),也可以是水分散在油中(W/O型)。分散相的 质点为球形,但半径非常小,通常为 10~100nm(0.01~0.1μm)范围。在相当 长的时间内,这种体系分别被称为亲水的油胶团(hydrophilic oleomicelles) 和亲油的水胶团(oleophilic hydromicelles),亦称为溶胀的胶团或增溶的胶 团。直到 1959 年,Schulman等人才首次将上述体系称为“微乳状液”或“微 乳液(microemulsion)。于是“微乳液”一词正式诞生[2]。
烷基酸的表面活性剂性质
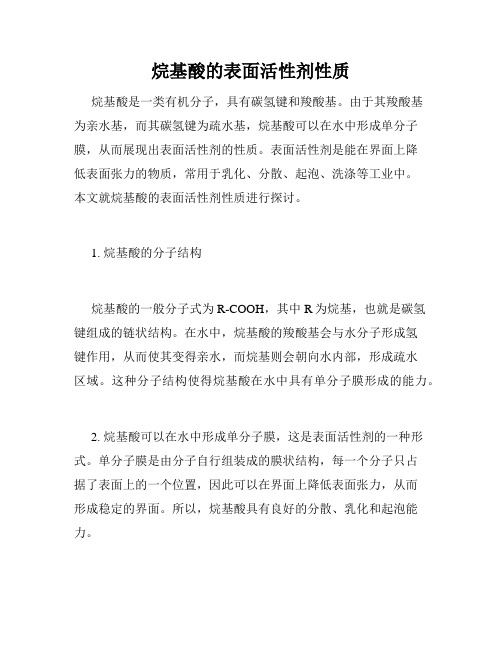
烷基酸的表面活性剂性质烷基酸是一类有机分子,具有碳氢键和羧酸基。
由于其羧酸基为亲水基,而其碳氢键为疏水基,烷基酸可以在水中形成单分子膜,从而展现出表面活性剂的性质。
表面活性剂是能在界面上降低表面张力的物质,常用于乳化、分散、起泡、洗涤等工业中。
本文就烷基酸的表面活性剂性质进行探讨。
1. 烷基酸的分子结构烷基酸的一般分子式为R-COOH,其中R为烷基,也就是碳氢键组成的链状结构。
在水中,烷基酸的羧酸基会与水分子形成氢键作用,从而使其变得亲水,而烷基则会朝向水内部,形成疏水区域。
这种分子结构使得烷基酸在水中具有单分子膜形成的能力。
2. 烷基酸可以在水中形成单分子膜,这是表面活性剂的一种形式。
单分子膜是由分子自行组装成的膜状结构,每一个分子只占据了表面上的一个位置,因此可以在界面上降低表面张力,从而形成稳定的界面。
所以,烷基酸具有良好的分散、乳化和起泡能力。
在水的界面上,烷基酸的羧酸基会向水外部释放H+,而疏水的烷基则会相互聚集形成疏水区域。
这种聚集使得烷基酸的表面张力变得较小,从而可以稳定地存在于界面上并降低表面张力。
此外,在烷基酸分子与水分子之间形成氢键作用时,可以吸收或释放能量,因此,烷基酸还具有减缓表面活性剂吸收能量的作用。
3. 烷基酸用作洗涤剂烷基酸的表面活性剂性质使其适用于洗涤剂的制造。
由于其具有非离子表面活性剂和阳离子表面活性剂的性质,因此可以用于洗涤剂的乳化和起泡作用,同时还可以作为缓冲剂,使得洗涤剂具有稳定性。
此外,烷基酸还具有卓越的去污性能,可以有效地清洗衣物、餐具和地板等。
4. 烷基酸的环境影响然而,在使用烷基酸洗涤剂时,也需要注意到它的环境影响。
烷基酸的排放会导致水体富营养化和水藻的暴发,从而影响水质和水体生态系统。
因此,在使用烷基酸洗涤剂时,应注意合理使用和妥善处理废水,以减少对环境的污染。
总之,烷基酸作为表面活性剂具有良好的分散、乳化和起泡能力,并被广泛应用于洗涤剂等工业领域。
烷基硫酸的表面活性剂性质
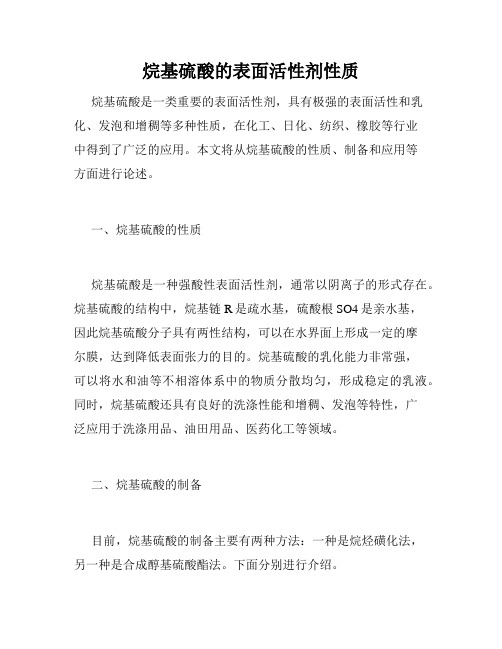
烷基硫酸的表面活性剂性质烷基硫酸是一类重要的表面活性剂,具有极强的表面活性和乳化、发泡和增稠等多种性质,在化工、日化、纺织、橡胶等行业中得到了广泛的应用。
本文将从烷基硫酸的性质、制备和应用等方面进行论述。
一、烷基硫酸的性质烷基硫酸是一种强酸性表面活性剂,通常以阴离子的形式存在。
烷基硫酸的结构中,烷基链R是疏水基,硫酸根SO4是亲水基,因此烷基硫酸分子具有两性结构,可以在水界面上形成一定的摩尔膜,达到降低表面张力的目的。
烷基硫酸的乳化能力非常强,可以将水和油等不相溶体系中的物质分散均匀,形成稳定的乳液。
同时,烷基硫酸还具有良好的洗涤性能和增稠、发泡等特性,广泛应用于洗涤用品、油田用品、医药化工等领域。
二、烷基硫酸的制备目前,烷基硫酸的制备主要有两种方法:一种是烷烃磺化法,另一种是合成醇基硫酸酯法。
下面分别进行介绍。
1、烷烃磺化法烷烃磺化法是一种常见的制备烷基硫酸的方法,其原理是将烷烃分子的烷基链上的氢原子取代为磺酸基。
通常使用浓硫酸和氯磺酸等作为磺化试剂,在高温条件下反应。
烷烃磺化反应可以分为两步,首先是烷烃和磺酸的加成反应,生成磺酸化的烃烷基磺酸,然后再进行酸解,生成所需的烷基硫酸。
2、合成醇基硫酸酯法合成醇基硫酸酯法是一种相对新颖的烷基硫酸的制备方法,其原理是采用工业丙烯酸解氨法生产的丙烯醇为原料,通过硫酸催化加成反应生成醇基硫酸酯,然后经过硫酸钠水解得到所需的烷基硫酸。
这种方法可以避免使用有毒的磺化试剂,生产环境更加安全。
三、烷基硫酸的应用烷基硫酸作为一种非常重要的表面活性剂,具有非常广泛的应用领域。
下面列举几个主要的应用领域进行介绍。
1、洗涤用品洗涤用品是烷基硫酸最主要的应用领域之一,主要包括洗衣粉、洗洁精、洗碗精、洗手液等多种产品。
烷基硫酸作为洗涤用品中的主要表面活性剂,具有良好的去污能力和稳定的泡沫性能,在清洁效果和使用体验方面都得到了广泛的认可。
2、油田用品烷基硫酸也是一种非常重要的油田用品原料,主要用于油井酸化和清洗、防腐等方面。
烷基磺酸的表面活性剂性质
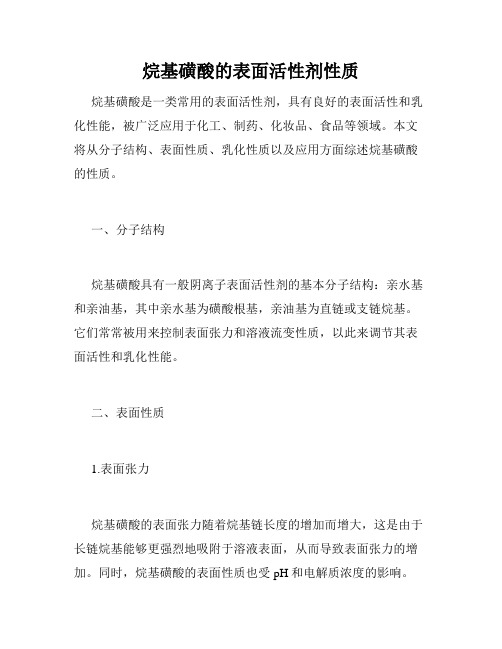
烷基磺酸的表面活性剂性质烷基磺酸是一类常用的表面活性剂,具有良好的表面活性和乳化性能,被广泛应用于化工、制药、化妆品、食品等领域。
本文将从分子结构、表面性质、乳化性质以及应用方面综述烷基磺酸的性质。
一、分子结构烷基磺酸具有一般阴离子表面活性剂的基本分子结构:亲水基和亲油基,其中亲水基为磺酸根基,亲油基为直链或支链烷基。
它们常常被用来控制表面张力和溶液流变性质,以此来调节其表面活性和乳化性能。
二、表面性质1.表面张力烷基磺酸的表面张力随着烷基链长度的增加而增大,这是由于长链烷基能够更强烈地吸附于溶液表面,从而导致表面张力的增加。
同时,烷基磺酸的表面性质也受pH和电解质浓度的影响。
2.胶束形成在溶液中,烷基磺酸分子会形成胶束结构。
当烷基链长度较短时,烷基磺酸分子的胶束直径较小,而随着烷基链长度的增加,胶束大小逐渐增大。
同时,pH和电解质浓度也会影响胶束结构。
3.吸附特性烷基磺酸具有化学吸附和物理吸附两种吸附方式。
化学吸附是指烷基磺酸与表面形成化学键,而物理吸附则是烷基磺酸的疏水基与表面形成范德华力,二者都与表面张力和溶液流变性质密切相关。
三、乳化性质烷基磺酸在水中形成乳液时,由于其构成胶束结构的能力,因此可有效地改善油水相的乳化性质。
此外,烷基磺酸的乳化性质还受到乳化温度、电解质浓度和pH值等因素的影响。
四、应用方面烷基磺酸具有广泛的应用,主要在以下几个方面:1.化工领域烷基磺酸被用于工业清洗、油墨和颜料的乳化以及染料的分散等。
2.制药领域烷基磺酸可以用作某些制药品质的改进剂,如用于改善药物的生物利用度和增强药物的口服吸收等。
3.化妆品领域烷基磺酸可以作为某些化妆品(如洗发水、肥皂和香波)中的表面活性剂和乳化剂。
4.食品领域烷基磺酸可以用于增加食品的黏性、乳化和分散性,从而改善某些食品的口感和观感。
总之,烷基磺酸作为一种大众性的表面活性剂,其性质和应用十分广泛。
在不断地发展和创新中,它将继续为各个领域的生产和生活带来更多的便利和机遇。
- 1、下载文档前请自行甄别文档内容的完整性,平台不提供额外的编辑、内容补充、找答案等附加服务。
- 2、"仅部分预览"的文档,不可在线预览部分如存在完整性等问题,可反馈申请退款(可完整预览的文档不适用该条件!)。
- 3、如文档侵犯您的权益,请联系客服反馈,我们会尽快为您处理(人工客服工作时间:9:00-18:30)。
A family of alkyl sulfate gemini surfactants.1.Characterization of surface propertiesBo Gao ⇑,Mukul M.SharmaDepartment of Petroleum and Geosystems Engineering,The University of Texas at Austin,Austin,TX 78712,United Statesa r t i c l e i n f o Article history:Received 24February 2013Accepted 25April 2013Available online 3May 2013Keywords:Sulfate gemini surfactants Critical micelle concentration Electrical conductivity Surface tension MicellizationSurface adsorptiona b s t r a c tThe fundamental aqueous and surface properties of a family of sulfate gemini surfactants have been char-acterized.The critical micelle concentrations (cmc)were determined by both electrical conductivity and surface tension methods.The cmc values were found to be two orders of magnitude lower than those measured for single tail surfactants.The cmc values depend primarily on the surfactant tail length,and relatively little on the spacer length and solution temperature.The surface tension measurements suggest that current family of gemini surfactants have higher tendency to spontaneously adsorb at the air–water interface and thus are much more efficient in reducing surface tension than conventional sin-gle-chain surfactants.Thermodynamic calculations of Gibbs free energies for micellization and adsorp-tion indicate surface adsorption is promoted more than micellization for these sulfate gemini surfactants.This type of molecules may therefore be very efficient and cost-effective in applications that require ultra-low interfacial tensions and high interfacial activities.Ó2013Elsevier Inc.All rights reserved.1.IntroductionGemini surfactants represent a class of surfactants made up of two amphiphilic moieties connected at or very close to,the head groups by a spacer group [1].The current interest in such surfac-tants arises from their distinct properties [1–6].First and foremost,gemini surfactants have critical micelle concentration (cmc)values that are several orders of magnitude lower than those of the corre-sponding conventional (monomeric)surfactants.These molecules are also more efficient and effective in reducing the surface/inter-facial tension.Moreover,aqueous solutions of some gemini surfac-tants exhibit strong viscosifying capability even at relatively low concentration [4–6].While there have been many papers studying the aforemen-tioned properties for cationic gemini surfactants,very few reports deal with the surface and aqueous properties of anionic gemini surfactants [7–11],which are of particular interest in the energy sector [12].Unfortunately,at present there is not enough pub-lished information/data to establish structure performance rela-tionships for anionic geminis.It is thus important to look into anionic gemini surfactants of potential interest and study their rel-evant properties for practical applications.In the current study,a family of sulfate gemini surfactants was prepared.The solution and surface properties were systematically investigated using elec-trical conductivity and surface tension measurements.2.Experimental 2.1.MaterialsA family of seven alkyl sulfate gemini surfactants was synthe-sized in our group,following a two-step reaction scheme proposed by Rist and Carlsen [13].To the best of our knowledge,the funda-mental surface properties of these molecules have not been fully characterized,not to mention any application specific properties.The general structure of the synthesized gemini surfactants is illus-trated in Fig.1.As a general feature,the synthesized molecules contain double chains each consisting of hydrophobic alkyl chains that are terminated by ethylene sulfate (CH 2CH 2O–SO 3Na)head groups.The chains are interconnected by alkyloxy spacer groups.The surfactants are named ‘m –s –m ’,where m and s represent the number of carbon atoms in the tail and spacer groups of the mol-ecule (m =14,16,18and 20+;s =2and 4).Note here m of 20+rep-resents a mixture of surfactants with chain lengths ranging from 20to 30(by starting with a C 20–C 30epoxide mixture).All products exhibited spectroscopic properties that were in agreement with those expected for the desired structures.Synthe-sis scheme of current family of sulfate gemini surfactants,and 1H NMR spectra are giving in the Supplementary material .2.2.MethodsAll of the gemini surfactant solutions were prepared by diluting a stock (concentrated)solution with ultrapure Milli-Q water (resis-tivity =18.2M O cm),and stirred on a magnetic stirrer at desired temperature for an hour.0021-9797/$-see front matter Ó2013Elsevier Inc.All rights reserved./10.1016/j.jcis.2013.04.043⇑Corresponding author.Present address:3319Mercer Street,URC-URC-SW204,Houston,TX 77027,United States.Fax:+17134316360.E-mail address:b.robert.gao@ (B.Gao).2.2.1.Electrical conductivityElectrical conductivities were measured using a digital conduc-tivity meter(OaktonÒECTestr11+)from Eutech Instruments.Mea-surements were carried out in a water bath set at desired temperature and repeated twice for every sample.To obtain critical micelle concentration(cmc)of gemini surfac-tants,the conductance of the solution was recorded at various con-centrations.And then the cmc was calculated as the intersection of linear parts in the dependence of conductivity versus surfactant concentration.The degree of ionization a was calculated as the ra-tio of slopes above and below the cmc in the plot.2.2.2.Surface tensionThe surface tensions of aqueous solutions of the gemini surfac-tants were measured with a ring tensiometer(CSC DuNouy Tensi-ometer)using the du Nouy ring technique.All measurements were carried out at lab temperature of25°C.Sets of measurements were taken at10min intervals until the change in surface tension was less than0.05mN mÀ1.The cmc and the surface tension at the cmc(c cmc)were determined from the breakpoint of the surface tension versus the logarithm of the concentration curve.The sur-face tension method can be used for checking the sample purity and the absence of a minimum is a good sign.3.Results and discussion3.1.Aqueous solubilityThe solubility of ionic surfactants is commonly characterized by the Krafft temperature T K,which is the minimum temperature at which surfactants form micelles.As described by Hato and Shinoda [14],aqueous solutions of all sulfate gemini surfactants were pre-pared at a controlled temperature of20°C and concentration of 1.0wt.%.Upon sufficient mixing,all solutions were visually exam-ined to be clear and parably low Krafft temper-atures were also reported for other gemini surfactants[7,15,16].It should emphasize here that only the range of T K(620°C)is deter-mined here by visual inspection method described above.More definitive and accurate estimate can be obtained using more quan-titative techniques including electrical conductivity and spectrophotometry.The low Krafft temperatures of these surfactants might be attributed to the hydrophilic nature of their spacer and head groups[17–19].The hydrophilic–lipophilic balance(HLB)of a sur-factant is a measure of the degree to which it is hydrophilic or lipo-philic.Stronger hydrophilic(or weaker lipophilic)characteristics of the molecule will promote its affinity with water molecules and might facilitate its solubility in aqueous environment.Table1 shows a comparison of calculated HLB numbers(using Becher’s method[20])between current sulfate geminis and several sodium alkylsulfate surfactants of equivalent chain length.It is clearly seen that gemini surfactants have much higher HLB numbers and thus exhibit better aqueous solubility,compared to their conventional counterparts.3.2.Conductivity dataThe cmc of the surfactants was taken as the concentration at the intersection of the linear portions of the conductivity versus con-centration plots above and below the breakpoint,according to the Williams method[21],as shown in Fig.2for the single-chain surfactant sodium dodecyl sulfate(SDS,from Sigma–Aldrich)and in Fig.3for the gemini surfactant14–2–14at three different tem-peratures(30°C,40°C and60°C).As expected,the slope above the cmc is lower than the one below the cmc,as micelles are worse charge carriers when compared to surfactant monomers.The average degree of micellar ionization,a,was taken as theratio of the slopes above and below the cmc in the electrical con-ductivity versus concentration plots.The standard Gibbs energy of micellization(per mole of surfactant molecule),D G oM,for gemini surfactants with monovalent counterions can be calculated using (derivation provided in Supplementary material):D G oM¼2ð1:5ÀaÞRT ln cmcþ2ð1ÀaÞRT ln2ð1ÞMoreover,from the dependency of cmc and a to absolute tem-perature,the standard enthalpy and entropy of micellization D H oM and D S oMcan be estimated from(see Supplementary material):D H oM¼À2ð1:5ÀaÞRT2@lnðcmcÞ@Tð2ÞD S oM¼D H oMÀD G oMð3Þwhere R is the gas constant and T is absolute temperature.The micellar parameters obtained from conductivity data are summa-rized in Table2.The cmc,a and D G o M values for SDS obtained from current study are in good agreement with those reported in the literatureGeneral chemical structure of sulfate gemini surfactants investigated study.Table1HLB values for gemini and conventional surfactants.Gemini surfactant HLB Conventional surfactant HLB14–2–1473.45C12–SO4Na40.00 18–2–1872.50C14–SO4Na39.05 14–4–1469.65C16–SO4Na38.10 16–4–1668.70C18–SO4Na37.15 18–4–1864.90and Interface Science404(2013)80–8481[22–25],demonstrating the accuracy of our measurements.Forboth surfactants,D G oM remains negative while D S oMremains positiveover the temperature range investigated,confirming that micelleformation occurs spontaneously when the surfactant concentra-tion reaches the cmc value.The magnitudes of the energy terms for gemini surfactant14–2–14are in line with reported values for some cationic gemini sur-factants[26].The significantly more negative D G oMvalue for14–2–14surfactant(as compared to values for SDS)is the driving force for its micellization.The ultralow cmc is a direct result of the large negative free energy.Micellization of14–2–14is primarily an en-tropy driven process,whereas SDS experiences a transition from entropy driven to enthalpy driven as solution temperature in-creases.As can be seen in Table2,the entropy term,ÀT D S oM,dom-inates over the enthalpy term,D H oM,over a wide temperature range.On the other hand,for SDS the dominant energy term switches from entropy to enthalpy at midpoint of the temperature range.This is intuitively understandable considering the much lar-ger disruption to the water structure(entropic effect)caused by double tail groups from a gemini surfactant.Table3above summarizes all the cmc values for the current family of sulfate gemini surfactants,compared with values for three single-chain surfactants.The cmc values for gemini surfac-tants are two to three orders of magnitude lower than those for the conventional single-tail molecule.These cmc measurements fully demonstrate the strong tendency of gemini surfactants to self-aggregate and form micellar structures in aqueous solutions at ultralow concentrations.Moreover,the cmc values depend pri-marily on the surfactant tail chain length,and relatively little on the length of spacer and solution temperature.Fig.4shows the plots of the cmc logarithms against the alkyl chain length,m.A fairly linear relationship was observed,with R2determined to be0.9740and0.9556for the m–2–m and m–4–m series respectively.In general,an empirical equation that relates the cmc to surfac-tant alkyl chain length can be expressed as[27]:log cmc¼AÀBmð4Þwhere A is a constant for a particular homologous series,and B rep-resents the energy contribution of the methylene group[28].The B values were estimated to be0.0999and0.1013for m–2–m and m–4–m,respectively,which were smaller than most reported values for cationic gemini surfactants($0.430)[2].This indicates that sul-fate gemini micelles have a comparatively weaker cohesive force(or stronger electrical repulsive force).3.3.Surface tension dataFrom surface tension measurements the cmc and the surface tension at the cmc were determined from the breakpoint in the surface tension versus logarithm of surfactant concentration pro-file,as exemplified in Figs.5and6for the gemini surfactants stud-ied(m–2–m and m–4–m series respectively).The maximum surface excess,C max,at the air-solution interface and the minimum area per molecule,a min,were calculated by applying the Gibbs adsorption isotherm[29],C max¼À12:303nRTd cd log Cmaxð5ÞTable2Critical micelle concentration(cmc),degree of micellar ionization(a)and standardenergies of micellization(D G oM ;D H oMandÀT D S oM)obtained from conductivity data atdifferent temperatures.Surfactant T(°C)cmc(l mol LÀ1)a D G oM(kJ molÀ1)D H oM(kJ molÀ1)ÀT D S o M(kJ molÀ1)SDS308.30Â1030.2192À21.49À4.64À16.85 408.63Â1030.2415À21.75À8.63À13.126010.28Â1030.2623À22.02À16.92À5.1014–2–1430 5.140.1591À79.43À22.50À56.8740 6.020.1710À80.21À29.52À50.70607.990.1852À82.35À44.05À38.30Table3CMC values for the family of anionic gemini surfactants.Surfactant cmc(l mol LÀ1)30°C40°C60°C14–2–14 5.14 6.027.9918–2–18 1.53 1.78 2.3020+–2–20+0.620.90 1.2314–4–14 4.79 5.10 5.4616–4–16 2.28 2.53 2.9118–4–18 1.22 1.44 1.8020+–4–20+0.540.570.62C12–SO4Na8.22Â1038.63Â10310.24Â103 C14–SO4Na 2.13Â103 2.19Â103 2.80Â103 C16–SO4Na–0.59Â103–82 B.Gao,M.M.Sharma/Journal of Colloid and Interface Science404(2013)80–84a min ¼1016N A v C maxð6Þwhere c is the surface tension in mN m À1,R is the gas constant (8.314J mol À1K À1),T is the absolute temperature,N Av is the Avoga-dro’s number,C max is in mol cm À2,and a min is the in Å2.For gemini surfactants,various investigators have used 2or 3for n [1].In all calculations that follow,n value of 2will be used based on the understanding that it is merely indicative of the change in C max within the family of compounds.A convenient measure of the efficiency of adsorption is the neg-ative logarithm of the concentration of surfactant in the bulk phase required to produce a 20mN m À1reduction in surface tension:p C 20=Àlog C 20.p C 20can be calculated using the following relation [29]:pC 20¼Àlog C 20¼p cmc À202:303nRT C maxÀlog cmc ð7Þwhere p cmc is the surface pressure defined by p cmc ¼c 0Àc cmc .The standard Gibbs free energy of adsorption D G o ad tells us whether adsorption is spontaneous or not and the magnitude of the driving force:D G o ad ðin J =mol Þ¼2:303nRT log ðC p =x ÞÀ6:023p a minð8Þwhere C p is the molar concentration of surfactant in the aqueous phase at a surface pressure of p cmc and x is moles of water per liter.Micellar parameters obtained from surface tension measurements are summarized in Table 4.The cmc values obtained from surface tension data agree well with the ones obtained from conductivity measurements.The small differences may be related with specifics of the methods used,since surface tension measurements are sensitive primarily to the surfactant monomers,as micelles are not generally thought to be surface active,while electrical conductivity method depends on the conductivity of all the ionic species present.Also seen from the table is the fact that micellization of gemini surfactants takes place at a concentration below 10l mol L À1,which is about three orders of magnitude lower than that of SDS.The extraordinary ability to self-aggregate is an important property of gemini surfac-tants.Lower p cmc values are also observed.For ionic surfactant molecules in water,the micelle formation is a direct result of two opposing forces,an attractive force favoring aggregation and closer packing and an electrostatic repulsive force that prevents larger size micelles.The attractive force arises from the hydrophobic effect [30]acting upon the hydrocarbon chains of the surfactants.The hydrophobic effect is primarily an entropic effect [31]originating from the disruption of highly dynamic hydrogen bonds between molecules of liquid water by the hydro-carbon chains.The repulsive force in micelle formation comes pri-marily from electrostatic interactions between the head groups.Longer hydrocarbon tails will thus induce stronger hydrophobic attraction which results in a tighter packing and lower surface ten-Table 4Critical micelle concentration (cmc),surface tension at the cmc (c cmc ),saturation adsorption (C max ),area per molecule at interface (a min ),p C 20,cmc/C 20,and standard Gibbs adsorption energy obtained from surface tension data at 25°C.Surfactant cmc (l mol L À1)c cmc (mN m À1)C max Â1010(mol cm À2)a min (Å2)p C 20cmc/C 20D G o ad (kJ mol À1)14–2–14 5.2833.70 2.1379 5.99 5.22À99.3618–2–18 1.7326.53 3.5048 6.39 4.14À100.1920+–2–20+0.6820.87 5.0133 6.70 3.33À102.0014–4–14 4.9232.59 1.9884 6.12 6.47À101.4116–4–16 2.2028.02 2.2872 6.537.38À104.7118–4–18 1.0424.00 3.4349 6.70 4.98À103.9620+–4–20+0.5318.34 4.11417.00 5.01À106.47SDS8.54Â10338.733.08532.422.22À54.70Table 5Standard free energy of micellization and adsorption for gemini surfactants studied.Surfactant D G o M (kJ mol À1)D G o ad (kJ mol À1)14–2–14À79.43À99.3618–2–18À90.61À100.1920+–2–20+À97.68À102.0014–4–14À78.54À101.4116–4–16À84.97À104.7118–4–18À90.82À103.9620+–4–20+À97.07À106.47B.Gao,M.M.Sharma /Journal of Colloid and Interface Science 404(2013)80–8483sion at an air–water interface,provided that the electrostaticrepulsion remains unchanged.Since surface tension reduction depends on the replacement ofwater molecules at the interface by surfactant molecules,the effi-ciency(e.g.p cmc and p C20)of a surfactant should reflect the inter-facial surfactant concentration relative to that in the bulk liquidphase.The ratio of surfactant concentration at surface to that inbulk phase,C S/C,is,therefore,a suitable measure.The surface con-centration C S(in mole LÀ1)in turn can be related to the surface ex-cess concentration C(in mole cmÀ2)by the relation C S%1000C/d, where d(in cm)is the thickness of surface/interfacial region.C S/ C=1000C/C d.When the tension has been reduced by20mN mÀ1, C is close to C max,and most surfactant molecules are lying tilted at the interface[32].Assuming that the thickness of the interfacial re-gion d is determined by the height of surfactant normal to the interface,then d is inversely proportional to the minimum surface area per molecule a min.A larger value of a min generally indicates a smaller angle of the surfactant with respect to the interface;a smaller value of a min indicates an orientation of the surfactant more perpendicular to the interface.All the above discussions explain why we see a general trend ofdecreasing a min,increasing C max,and resulted decreasing p cmc as the hydrocarbon chain of geminis becomes longer.The much high-er p C20values of gemini surfactants over SDS represent the supe-rior efficiency of gemini molecules in reducing surface tension. The cmc/C20ratios decease as the alkyl chain length increases.The standard free energies of adsorption D G oadfor all gemini surfac-tants are negative;indicating that adsorption of these compoundsat the air–water interface is spontaneous.The values of D G oM and D G oadare compared side by side in Table5.The negative values of both free energy terms illustrate once againthe great potential for gemini surfactants to form micelles in solu-tion and to adsorb onto the air–water interface.With D G oMshowingconsistently smaller magnitudes than D G oad,current dataset sug-gests adsorption is energetically favored over micellization for this family of surfactants.This result is supported by the large values of p C20reported above.4.ConclusionsIn this article,we prepared a family of alkyl sulfate gemini sur-factants with systematically changing structures and investigated their fundamental properties such as their cmc,surface tension, and adsorption/micellization parameters in order to understand their structures and performance relationships.With two hydro-philic head groups introduced into the structure,they showed high water solubility.The relationship between the cmc and hydrocar-bon chain lengths gave fairly linear trends,similar to what would typically be observed for conventional surfactants[27],despite the double head double tail structure.It is worth emphasizing that the cmc values of the sulfate gemini surfactants were orders of magnitude lower than those of single chain surfactants with the same alkyl chain length.All the surfactants studied show great effi-ciency in surface tension reduction and are more likely to adsorb at the air/water interface rather than to form micelles,a behavior also found for some other anionic gemini surfactants[33,34].Until now,although a large number of novel surfactants have been designed and synthesized by many researchers[2,35],to the best of our knowledge,a systematic study of structure surface/aqueous property relationships of the titled anionic gemin-is,with clear end use in the energy sector[12],have not been re-ported.Therefore,the current article serves to set the stage for subsequent studies focused on more application oriented topics: the water–oil interfacial tension reduction potential of titled sul-fate geminis will be reported in a subsequent paper;their rheolog-ical properties in solution will be the subject of further study. AcknowledgmentsInsightful inputs from Dr.Fredric M.Menger(Emory Univer-sity),Dr.Lei Shi(Princeton University),Dr.Gary A.Pope and Dr. Upali P.Weerassoriya(The University of Texas at Austin)are grate-fully acknowledged.Appendix A.Supplementary materialSupplementary data associated with this article can be found,in the online version,at /10.1016/j.jcis.2013.04.043. References[1]M.J.Rosen,ChemTech(1993)30.[2]R.Zana,J.Xia(Eds.),Gemini Surfactants,Synthesis,Interfacial and Solution-Phase Behavior,and Applications,Marcel Dekker,New York,2003(Chapter1).[3]F.M.Menger,C.A.Littau,J.Am.Chem.Soc.115(1993)10082.[4]F.M.Menger,J.S.Keiper,Angew.Chem.Int.Ed.39(2000)1906.[5]R.Zana,J.Colloid Interface Sci.248(2002)203.[6]D.Danino,Y.Talmon,R.Zana,Langmuir11(1995)1448.[7]Y.-P.Zhu,A.Masuyama,M.Okahara,J.Am.Chem.Soc.67(1990)459.[8]S.Magdassi,M.M.Ben,Y.Talmon,D.Danino,Colloids Surf.A212(2003)1.[9]X.Du,Y.Lu,L.Li,J.Wang,Z.Yang,Colloids Surf.A290(2007)132.[10]T.Yoshimura,K.Esumi,J.Colloid Interface Sci.276(2004)231.[11]S.Tai,Z.Gao,X.Liu,Q.Zhang,Eru.J.Lipid Sci.Technol.114(2012)1062.[12]B.Gao,M.M.Sharma,Paper SPE159700,Presented at the SPE Annual TechnicalConference and Exhibition,San Antonio,Texas,October8–10,2012.[13]O.Rist,P.H.J.Carlsen,m.29(1999)749.[14]M.Hato,K.Shinoda,J.Phys.Chem.77(1973)378.[15]Y.-P.Zhu,A.Masuyama,Y.Kirito,M.J.Okahara,J.Am.Chem.Soc.68(1991)539.[16]Y.-P.Zhu,A.Masuyama,Y.Kirito,M.Okahara,M.J.Rosen,J.Am.Chem.Soc.69(1992)626.[17]M.Raison,Angew.Chem.69(1957)537.[18]T.Gu,J.Sjoblom,Acta Chem.Scand.45(1991)726.[19]T.Gu,J.Sjoblom,Colloids Surf.64(1992)39.[20]P.Becher,J.Disp.Sci.Technol.5(1985)81.[21]R.J.Williams,J.N.Phillips,K.J.Mysels,Trans.Faraday Soc.51(1955)728.[22]P.Mukerjee,K.J.Mysels,Critical Micelle Concentrations of Aqueous SurfactantSystems,NIST Standard Reference Data,</data/nsrds/NSRDS-NBS361.pdf>.[23]H.Stache,Anionic Surfactants,Organic Chemistry,Marcel Dekker,New York,1995.[24]M.Benrraou,B.L.Bales,R.Zana,J.Phys.Chem.B107(2003)13432.[25]E.A.Aniansson,S.N.Wall,M.Almegren,J.Phys.Chem.80(1976)905.[26]A.B.Groswasser,R.Zana,Y.Talmon,J.Phys.Chem.B104(2000)4005.[27]H.B.Klevens,J.Phys.Colloid Chem.52(1948)130.[28]T.Okano,N.Egawa,M.Fujiwara,M.Fukuda,J.Am.Oil Chem.Soc.73(1996)31.[29]M.J.Rosen,Surfactants and Interfacial Phenomena,3rd ed.,John Wiley andSons,New York,2004.[30]C.Tanford,The Hydrophobic Effect:Formation of Micelles and BiologicalMembranes,2nd ed.,John Wiley and Sons,New York,1973.[31]T.P.Silverstein,c.75(1998)116.[32]H.B.Aguiar,M.L.Strader,A.G.Beer,S.Roke,J.Phys.Chem.B115(2011)2970.[33]M.R.Infante,L.Perez,A.Pinazo,P.Clapes,M.C.Moran,M.Angelet,M.T.Garcia,M.P.Vinardell,C.R.Chim.7(2004)583.[34]C.M.C.Faustino,A.R.T.Calado,L.Garcia-Rio,Biomacromolecules10(2009)2508.[35]K.Holmberg(Ed.),Novel Surfactants,Preparation,Applications,andBiodegradability,2nd ed.,John Wiley and Sons,New York,2003.84 B.Gao,M.M.Sharma/Journal of Colloid and Interface Science404(2013)80–84。