ASME动力管道
管道编号规则
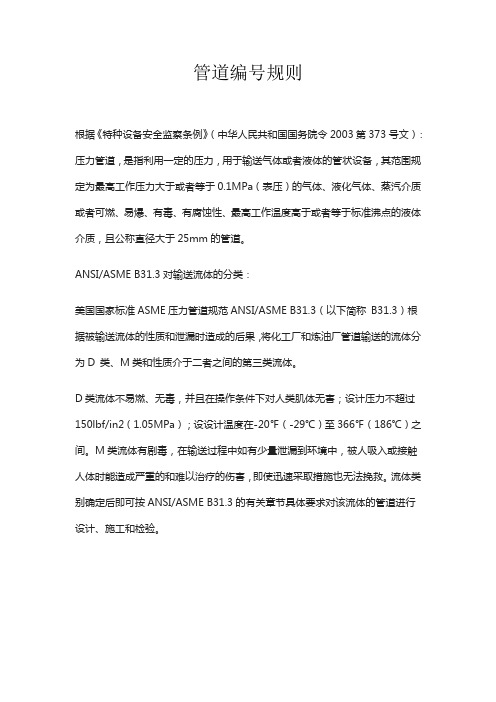
管道编号规则根据《特种设备安全监察条例》(中华人民共和国国务院令2003第373号文):压力管道,是指利用一定的压力,用于输送气体或者液体的管状设备,其范围规定为最高工作压力大于或者等于0.1MPa(表压)的气体、液化气体、蒸汽介质或者可燃、易爆、有毒、有腐蚀性、最高工作温度高于或者等于标准沸点的液体介质,且公称直径大于25mm的管道。
ANSI/ASME B31.3对输送流体的分类:美国国家标准ASME压力管道规范ANSI/ASME B31.3(以下简称B31.3)根据被输送流体的性质和泄漏时造成的后果,将化工厂和炼油厂管道输送的流体分为D 类、M类和性质介于二者之间的第三类流体。
D类流体不易燃、无毒,并且在操作条件下对人类肌体无害;设计压力不超过150lbf/in2(1.05MPa);设设计温度在-20℉(-29℃)至366℉(186℃)之间。
M类流体有剧毒,在输送过程中如有少量泄漏到环境中,被人吸入或接触人体时能造成严重的和难以治疗的伤害,即使迅速采取措施也无法挽救。
流体类别确定后即可按ANSI/ASME B31.3的有关章节具体要求对该流体的管道进行设计、施工和检验。
压力管道分类:(根据TSGR1001-2008《压力容器压力管道设计许可规则》整理)1.GA类(长输管道)长输(油气)管道是指产地、储存库、使用单位之间的用于输送商品介质的管道,划分为GA1级和GA2级。
1)符合下列条件之一的长输管道为GA1级:(1)输送有毒、可燃、易爆气体介质,最高工作压力P>4.0MPa 的长输管道;(2)输送有毒、可燃、易爆液体介质,最高工作压力P≥6.4MPa,并且输送距离(指产地、储存地、用户间的用于输送商品介质管道的长度))≥200km的长输管道;2)GA1级以外的长输(油气)管道为GA2级。
GA1级分为GA1甲级、GA1乙级:1、GA1甲级(1)输送有毒、可燃、易爆气体或者液体介质的管道。
(2)输送有毒、可燃、易爆气体或者液体介质的管道。
ASME 压力管道规范B31.1领会要点
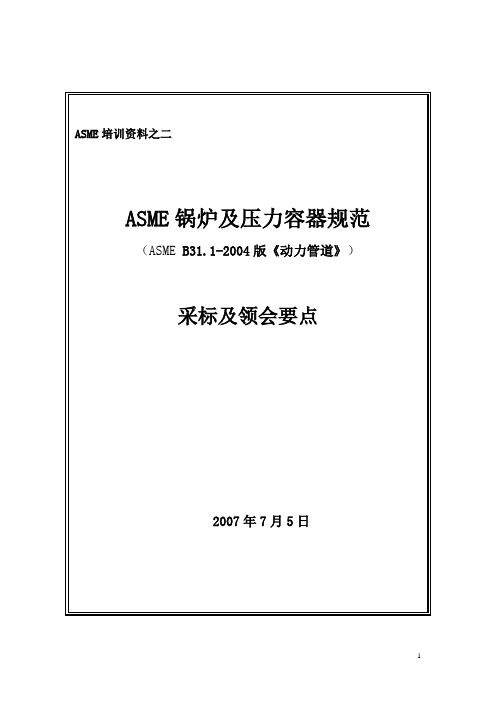
ASME 压力管道规范B31.1-2004版《动力管道》采标及领会要点ASME 第Ⅰ卷《动力锅炉建造规则》采标提示:基本范围:本卷对动力锅炉、电热锅炉和小型锅炉、固定式高温热水锅炉以及用于机车、移动作业和牵引作业的动力锅炉的全部制造过程提出要求。
另外,还包括V、A、M、PP、S和E规范标志钢印的使用规则。
本规范适用于压力大于15psig的蒸汽锅炉以及工作压力超过160psig和/或工作温度超过250°F的高温热水锅炉。
过热器、节热器和其他不经阀门而直接连接在锅炉上的压力部件均属于本规范的适用范围。
内容:上述要求适用于焊接锅炉;铆接锅炉(仅作参考);水管锅炉;火管锅炉;给水加热器、小型锅炉;电热锅炉;有机液体汽化器。
参照的锅炉与压力容器规范:ASME 第Ⅱ卷《材料》;第Ⅳ卷《供热锅炉》;第Ⅴ卷《无损检测》;第Ⅶ卷《推荐的动力锅炉保养指南》;第Ⅷ卷《压力容器》第1册;第Ⅸ卷《焊接和钎焊资格鉴定》。
引用的标准与规范:ASME B1.20.1《通用管螺纹》;B16系列标准阀门、法兰、接头和垫圈(B16.1、B16.3、B16.4、B16.5、B16.9、B16.11、B16.15、B16.20、B16.24、B16.25、B16.28、B16.34、B16.42);;B36.10《焊接钢管和无缝钢管》;B31.1《动力管道》;PTC25《减压装置》;QAI-1《授权检验资质鉴定》。
ASME压力管道规范B31.1《动力管道》采标及领会要点解析引言ASME B31.1是全球普遍认可的动力管道标准,是在ASME B31压力管道规范委员会指导下制订的。
管道标准分两大类,一类是管道系统标准,有B31.1~B31.33,常用的为B31.1、B31.2、B31.3、 B31.4 、B31.5、B31.8;另一类为管道组件标准,包括法兰、垫片、紧固件、阀门、接头等,从B16.1~ B16.49近50个标准。
B31.1动力管道:基于典型的发电站、工业和公共设施电厂、地热系统以及集中及区域供热和冷却系统的管道。
ASME B31标准在海外油田地面工程建设中的应用
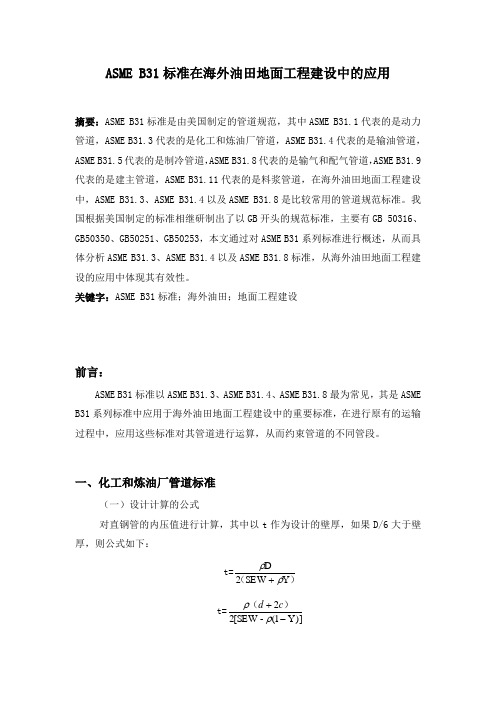
ASME B31标准在海外油田地面工程建设中的应用摘要:ASME B31标准是由美国制定的管道规范,其中ASME B31.1代表的是动力管道,ASME B31.3代表的是化工和炼油厂管道,ASME B31.4代表的是输油管道,ASME B31.5代表的是制冷管道,ASME B31.8代表的是输气和配气管道,ASME B31.9代表的是建主管道,ASME B31.11代表的是料浆管道,在海外油田地面工程建设中,ASME B31.3、ASME B31.4以及ASME B31.8是比较常用的管道规范标准。
我国根据美国制定的标准相继研制出了以GB 开头的规范标准,主要有GB 50316、GB50350、GB50251、GB50253,本文通过对ASME B31系列标准进行概述,从而具体分析ASME B31.3、ASME B31.4以及ASME B31.8标准,从海外油田地面工程建设的应用中体现其有效性。
关键字:ASME B31标准;海外油田;地面工程建设前言:ASME B31标准以ASME B31.3、ASME B31.4、ASME B31.8最为常见,其是ASME B31系列标准中应用于海外油田地面工程建设中的重要标准,在进行原有的运输过程中,应用这些标准对其管道进行运算,从而约束管道的不同管段。
一、化工和炼油厂管道标准(一)设计计算的公式对直钢管的内压值进行计算,其中以t 作为设计的壁厚,如果D/6大于壁厚,则公式如下: t=)(Y SEW 2Dρρ+ t=Y)]1(-SEW [22-+ρρ)(c dtm=t+c其中d以mm为单位值作为管道内径,通过以上公式对压力设计值进行计算,从而推断出设计厚度的公式:t s =YEjtρσρ+][2Dtsd= t8+CC=C1+ C2其中材料在设计温度下的许用应力用t][σ来表示,C1以及C2为附加量,只不过前者为厚度减薄的附加量,后者为复式的附加量,Y是在ASME B31.3基础上制定的GB 50316规定的系数,根据其不同情况下的系数标准从而进行内外径的计算,保证计算的壁厚符合应用标准。
ASME标准中文版
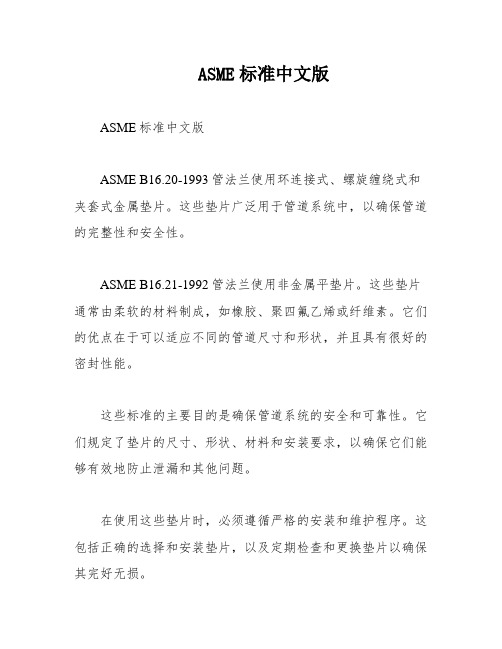
ASME标准中文版ASME标准中文版ASME B16.20-1993管法兰使用环连接式、螺旋缠绕式和夹套式金属垫片。
这些垫片广泛用于管道系统中,以确保管道的完整性和安全性。
ASME B16.21-1992管法兰使用非金属平垫片。
这些垫片通常由柔软的材料制成,如橡胶、聚四氟乙烯或纤维素。
它们的优点在于可以适应不同的管道尺寸和形状,并且具有很好的密封性能。
这些标准的主要目的是确保管道系统的安全和可靠性。
它们规定了垫片的尺寸、形状、材料和安装要求,以确保它们能够有效地防止泄漏和其他问题。
在使用这些垫片时,必须遵循严格的安装和维护程序。
这包括正确的选择和安装垫片,以及定期检查和更换垫片以确保其完好无损。
总之,ASME B16.20-1993和ASME B16.21-1992标准是管道系统中必不可少的组成部分。
它们确保了管道的安全和可靠性,并为管道系统的设计和维护提供了重要的指导。
XXX Code is a set of XXX and pressure vessels。
The Code is divided into several ns。
each of which XXX.n I of the XXX。
n。
n。
and testing of power boilers。
XXX materials。
welding。
and safety.n II of the XXX divided into several parts。
each of which covers a specific type of material used in the XXX ferrous materials。
while Part B covers non-XXX C covers welding rods。
wires。
and filler materials。
and Part D XXX.n III of the XXX of nuclear power plant components。
动力管道标准(一)
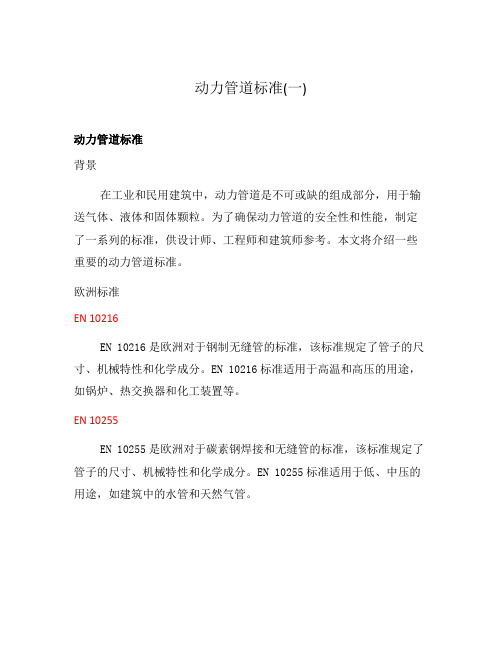
动力管道标准(一)动力管道标准背景在工业和民用建筑中,动力管道是不可或缺的组成部分,用于输送气体、液体和固体颗粒。
为了确保动力管道的安全性和性能,制定了一系列的标准,供设计师、工程师和建筑师参考。
本文将介绍一些重要的动力管道标准。
欧洲标准EN 10216EN 10216是欧洲对于钢制无缝管的标准,该标准规定了管子的尺寸、机械特性和化学成分。
EN 10216标准适用于高温和高压的用途,如锅炉、热交换器和化工装置等。
EN 10255EN 10255是欧洲对于碳素钢焊接和无缝管的标准,该标准规定了管子的尺寸、机械特性和化学成分。
EN 10255标准适用于低、中压的用途,如建筑中的水管和天然气管。
美国标准ASTM A106ASTM A106是美国对于无缝碳素钢管的标准,该标准规定了管子的尺寸、机械特性和化学成分。
ASTM A106标准适用于高温和高压的用途,如锅炉、热交换器和化工装置等。
ASME B31ASME B31是美国针对压力管道的标准,该标准覆盖了多种管道类型,如石油和天然气管道、化工管道和核电站管道等。
ASME B31标准规定了管道的设计、建造、测试和操作要求。
中国标准GB/T 8163GB/T 8163是中国对于无缝钢管的标准,该标准规定了管子的尺寸、机械特性和化学成分。
GB/T 8163标准适用于输送液体、气体和固体颗粒的用途,如石油、天然气和水管等。
GB 50235GB 50235是中国对于石油和天然气管道工程设计的标准,该标准规定了管道的设计、建设、测试和维护要求。
GB 50235标准适用于输送石油和天然气的长途管道工程。
总结以上介绍的标准只是动力管道标准中的一部分,此外还有其他的标准。
在设计、建造和使用动力管道时,要遵守相应的标准和法规,确保管道的安全性和性能。
常见问题1. 动力管道的设计标准有哪些?常见的设计标准包括:EN 10216、EN 10255、ASTM A106、ASME B31、GB/T 8163和GB 50235等。
ASME B31.1 and ASME B31.3的区别
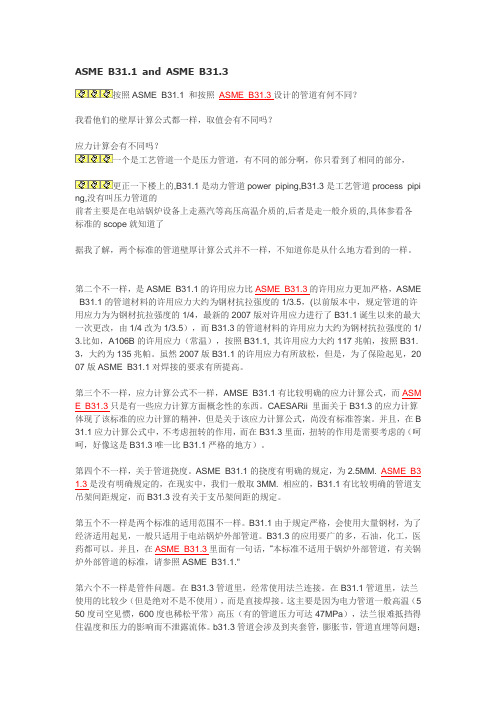
ASME B31.1 and ASME B31.3按照ASME B31.1 和按照ASME B31.3设计的管道有何不同?我看他们的壁厚计算公式都一样,取值会有不同吗?应力计算会有不同吗?一个是工艺管道一个是压力管道,有不同的部分啊,你只看到了相同的部分,更正一下楼上的,B31.1是动力管道power piping,B31.3是工艺管道process pipi ng,没有叫压力管道的前者主要是在电站锅炉设备上走蒸汽等高压高温介质的,后者是走一般介质的,具体参看各标准的scope就知道了据我了解,两个标准的管道壁厚计算公式并不一样,不知道你是从什么地方看到的一样。
第二个不一样,是ASME B31.1的许用应力比ASME B31.3的许用应力更加严格,ASME B31.1的管道材料的许用应力大约为钢材抗拉强度的1/3.5,(以前版本中,规定管道的许用应力为为钢材抗拉强度的1/4,最新的2007版对许用应力进行了B31.1诞生以来的最大一次更改,由1/4改为1/3.5),而B31.3的管道材料的许用应力大约为钢材抗拉强度的1/ 3.比如,A106B的许用应力(常温),按照B31.1, 其许用应力大约117兆帕,按照B31. 3,大约为135兆帕。
虽然2007版B31.1的许用应力有所放松,但是,为了保险起见,20 07版ASME B31.1对焊接的要求有所提高。
第三个不一样,应力计算公式不一样,AMSE B31.1有比较明确的应力计算公式,而ASM E B31.3只是有一些应力计算方面概念性的东西。
CAESARii 里面关于B31.3的应力计算体现了该标准的应力计算的精神,但是关于该应力计算公式,尚没有标准答案。
并且,在B 31.1应力计算公式中,不考虑扭转的作用,而在B31.3里面,扭转的作用是需要考虑的(呵呵,好像这是B31.3唯一比B31.1严格的地方)。
第四个不一样,关于管道挠度。
ASME B31.1的挠度有明确的规定,为2.5MM. ASME B3 1.3是没有明确规定的,在现实中,我们一般取3MM. 相应的,B31.1有比较明确的管道支吊架间距规定,而B31.3没有关于支吊架间距的规定。
压力管道设计常用ASME标准

一、压力管道设计常用ASME标准这里有两个标准,一个是组件尺寸型式标准(我国也有相应组件形式标准),另一个是材料标准(我国没有对材料形成专门的标准化)。
型式标准规定了组件的型式、系列、尺寸、公差、试验要求,以及该组件可采用的材料标准等。
材料标准规定了适用的对象、原材料(坯料)品种(采用锻轧Wrought或锻件Forged)、化学成分、机械性能、制造工艺(包括焊接)、热处理、无损检查、取样和性能检验、质量证书、标志等。
1. 典型的组件型式标准1)钢管ANSI/ASME B36.10M 无缝及焊接钢管ANSI/ASME B36.19M 不锈钢无缝及焊接钢管2)管件ANSI/ASME B16.9 工厂制造的钢对焊管件ANSI/ASME B16.1 承插焊和螺纹锻造管件ANSI/ASME B16.28 钢制对焊小半径弯头和回弯头3)阀门ANSI/ASME B16.34 法兰连接、螺纹连接和焊接连接的阀门API 599 法兰或对焊连接的钢制旋塞阀API 600 法兰或对焊连接的钢制闸阀API 602 紧凑型碳钢闸阀API 609 凸耳型对夹蝶阀4)法兰ANSI/ASME B16.5 管法兰和法兰管件ANSI/ASME B16.36 孔板法兰ANSI/ASME B16.42 球墨铸铁法兰和法兰管件ANSI/ASME B16.47 大直径钢法兰API 601 突面管法兰和法兰连接用金属垫片5)垫片ANSI/ASME B16.20 管法兰用缠绕式、包覆式垫片和环槽式用金属垫片ANSI/ASME B16.21 管法兰用非金属平垫片6)紧固件ANSI/ASME B18.2.1 方头和六角头螺栓和螺纹ANSI/ASME B18.2.2 方头和六角头螺母7)管件ASMEI B16.9 工厂制造的锻钢对焊管件ASME B16.11 承插焊和螺纹锻钢管件MSS-SP-43 锻制不锈钢对焊管件2. 材料标准ASTM/ASME材料标准主要集中收录在ASME II A篇铁基材料,B篇非铁基材料,C篇焊条、焊丝填充金属,D篇性能,以及一些增补内容。
压力管道审核-动力管道
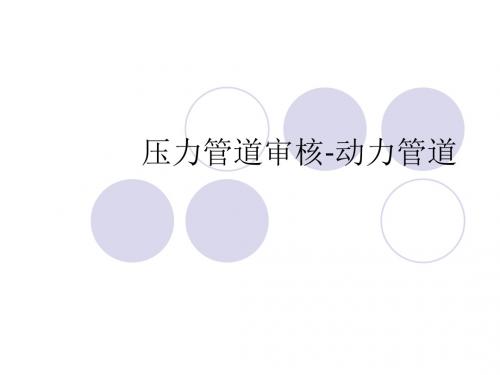
4.0设计参数
管子和管件的允许工作压力与公称压力可按下式
换算: t
[p]=PN——
s
式中[p]——允许的工作压力, MPa;
t——钢材在设计温度下的许用应力, MPa;
s——公称压力对应的基准应力,系指钢材在
200℃下的许用应力, MPa; PN—— 公称压力。
1.0 概述
1.3 火力发电厂主要汽水管道 火力发电厂主要汽水管道有:主蒸汽管道、
再热热段、再热冷段、高压给水、凝结水 等管道。
目录
1.0 概述 2.0 电厂管道设计常用标准 3.0 管道材料 4.0 设计参数 5.0 管道的选择 6.0 管道附件的选择 7.0 管道及附件的布置 8.0 管道的应力分析 9.0 管道支吊架的设计 10.0汽轮机防进水导则介绍 11.0 保温油漆
4.0设计参数
4.4 水压试验 管道安装完毕,必须对管道系统进行严密性检验。 水压试验用于检验管子和附件的强度及检验管系 的严密性。水压试验的压力(表压),应不小于 1.5倍的设计压力,且不得小于0.2MPa。水压试 验用水温度应不低于5℃,不大于70℃,环境温 度不得低于5℃。水压试验用水水质,必须清洁且 对管道系统材料的腐蚀性要小。对于奥氏体不锈 钢管道必须采用饮用水,且氯离子含量不超过 25mg/L。
压力管道审核-动力管道
目录
1.0 概述 2.0 电厂管道设计常用标准 3.0 管道材料 4.0 设计参数 5.0 管道的选择 6.0 管道附件的选择 7.0 管道及附件的布置 8.0 管道的应力分析 9.0 管道支吊架的设计 10.0汽轮机防进水导则介绍 11.0 保温油漆
允许偏差 DIN17175 高温用无缝钢管;采购技术条款 ASTM /ANSI B31.1 动力管道
ASME标准简介系列四压力管道
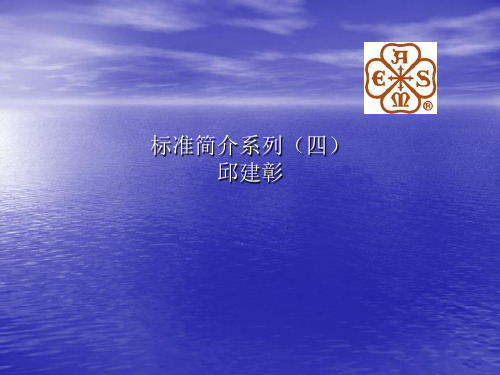
• 本规范适用的管道包括按表126.1 所列标准制造或专门设计的管子、
法兰、螺栓、垫圈、阀门、泄放装置、管配件和其它管道部件的承压 部位。也包括悬吊和支承件以及其它一些为防止承压零部件超压所必 需的设施。
• 各种其他管道仪表附件如水表柱、低读水位表、压力表、玻璃管水位
11111111111括压气机、调节站和计量器)间输送主要为气体产 11111111111品的管道以及集汽管道)
• 7)B31.9——房屋建筑用户管道(主要为工业设备、公共机构、
11111111111商业和市政建筑以及多单元住宅内和管道,但不包 11111111111括B31。1 所覆盖的尺寸、压力和温度范围)
• 4)B31.4——液态烃和其它液体的输送管线系统(工厂与终端设
11111111111备间以及终端设备、泵站、调节站和计量站内输送 11111111111主要为液体11产品的管道)
• 5)B31.5——冷冻管道(冷冻和二次冷却器的管道)
《压力管道标准规范B31》的组成
• 6)B31.8——气体输送和配汽管道系统(生产厂与终端设备(包
三、《 B31.1 动力管道》简介
《 B31.1 动力管道》简介
• B31.1 动力管道 • 本规范卷的内容包括: • (1) 可接受的材料技术条件和部件标准,包括尺寸要求111111和
压力—温度额定值的参考文献;
• (2) 包括管道支架的部件、组件设计要求; • (3) 随压力、温度变化和其它力作用对应力、反作用力111111和
所要求装设的阀门。
《 B31.1 动力管道》简介
– 锅炉本体终点处本身被认为是锅炉范围内管道的一部份, 终点与动力锅炉范围内管道见图100.1.2(A)、100.1.2(B)、 100.1.2(C)所示.。
ASME 中tube与pipe区别

ASME 锅炉及压力容器规范对tube, pipe, tubing和piping 这四个名词(术语)的含义是很严密的,适用于不同的场合。
现将我对四个名词的理解分述如下。
1、 tube 是圆形的,或具有连续周边的任何其他截面形状的空心制品。
圆形 tube 的尺寸可以用外径、内径、壁厚三者中的任意两个来指定。
8 N- A8 L! h; \ E& w" E s! i. O2、 pipe 是符合ASNI B36.10和B36.19(用于不锈钢)所列公称尺寸的圆形截面的tube , 它的直径用NPS号表示,NPS号与实际外径是不一致的,如NPS8 其外径是8.625 英寸。
管子壁厚用schedule No. 表示,同一NPS号可以有各种Sch. No.。
Sch. No. 有标准壁厚(STD)、加厚壁厚(XS)和特厚壁厚(XXS)之分等等。
0 m. \; N! f4 ]% p% m因此,tube 和 pipe 的基本差别是制造所依据的尺寸标准。
其次pipe 只有圆管,tube 可以有各种截面形状,pipe 只是tube 中的一个特例。
第三,无论tube 还是pipe 都是指材料生产厂生产出的原材料。
3、 tubing 是用tube 按PG-27.2.1[外径D≤5in.(127mm)]的计算公式计算后选定直径和厚度,并按设计要求加工(如弯管、开坡口、甚至油漆等)后制成的产品。
我们可以叫它管子件。
' J, N1 ?: }( i4、 piping 一般选用pipe 按PG-27.2.2的计算公式计算后选定NPS和Sch. No,并按设计要求加工后制成的产品,我们称作管道,但锅炉制造厂一般将这些管子叫做导管。
tubing 和piping的区别是1.选用的原材料不同,tubing 一般选用tube 加工制造piping 选用pipe 加工制造。
2. 计算用的公式不同。
在这里我们不讨论计算公式的差别。
ASME-BPE-标准解析
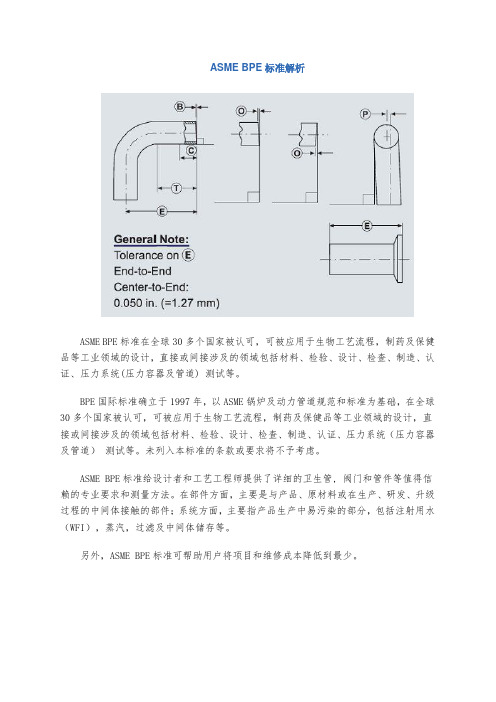
ASME BPE标准解析ASME BPE标准在全球30多个国家被认可,可被应用于生物工艺流程,制药及保健品等工业领域的设计,直接或间接涉及的领域包括材料、检验、设计、检查、制造、认证、压力系统(压力容器及管道) 测试等。
BPE国际标准确立于1997年,以ASME锅炉及动力管道规范和标准为基础,在全球30多个国家被认可,可被应用于生物工艺流程,制药及保健品等工业领域的设计,直接或间接涉及的领域包括材料、检验、设计、检查、制造、认证、压力系统(压力容器及管道)测试等。
未列入本标准的条款或要求将不予考虑。
ASME BPE标准给设计者和工艺工程师提供了详细的卫生管, 阀门和管件等值得信赖的专业要求和测量方法。
在部件方面,主要是与产品、原材料或在生产、研发、升级过程的中间体接触的部件;系统方面,主要指产品生产中易污染的部分,包括注射用水(WFI),蒸汽,过滤及中间体储存等。
另外,ASME BPE标准可帮助用户将项目和维修成本降低到最少。
ASME BPE的主要优势!严格控制部件硫含量在%~%之间, 以便在使用自动焊机焊接过程中补焊(见表DT-3, ASME BPE-2005)(见表SF-4,ASME BPE-2005)明确定量地规定了多种表面处理方式和表面粗糙系数。
在Ra平均值要求的基础上增加了表面粗糙系数Ra最大值。
(见表SF-4, ASME BPE-2005)规定了测量和公差的要求。
(见表DT-4, ASME BPE-2005)定量地规定了如何测量不同种类, 由不同原因引起的瑕疵,如原材料质量、机械工艺、焊接瑕疵等。
(见表 SF-3, ASME BPE-2005)明确规定了零部件的标识和包装的标准。
(见表DT-3, ASME BPE-2005)明确规定了“最大压力等级”。
(见表DT-2, ASME BPE-2005)!精确规定了系统和元件的基本概念。
(见表GR-10, ASME BPE-2005)对卫生及无菌零部件和各类管道元件设计有了总体要求, 例如: 阀,泵及换热器。
ASME Ⅲ管道设计
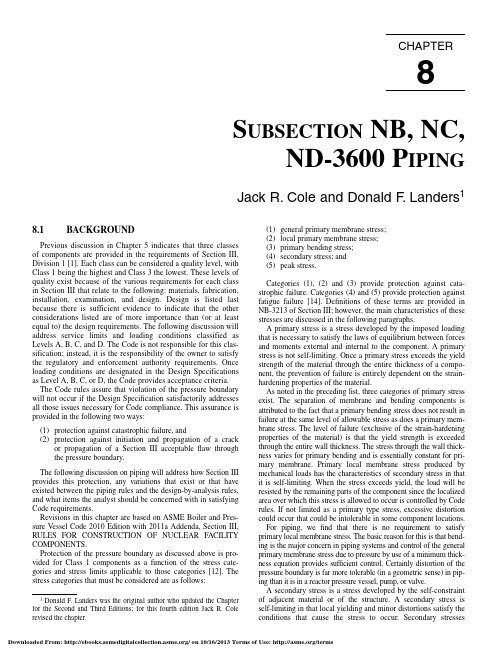
8.1BACKGROUNDPrevious discussion in Chapter 5 indicates that three classes of components are provided in the requirements of Section III, Division 1 [1]. Each class can be considered a quality level, with Class 1 being the highest and Class 3 the lowest. These levels of quality exist because of the various requirements for each class in Section III that relate to the following: materials, fabrication, installation, examination, and design. Design is listed last because there is sufficient evidence to indicate that the other considerations listed are of more importance than (or at least equal to) the design requirements. The following discussion will address service limits and loading conditions classified as Levels A, B, C, and D. The Code is not responsible for this clas-sification; instead, it is the responsibility of the owner to satisfy the regulatory and enforcement authority requirements. Once loading conditions are designated in the Design Specifications as Level A, B, C, or D, the Code provides acceptance criteria. The Code rules assure that violation of the pressure boundary will not occur if the Design Specification satisfactorily addresses all those issues necessary for Code compliance. This assurance is provided in the following two ways:(1)protection against catastrophic failure, and(2)protection against initiation and propagation of a crackor propagation of a Section III acceptable flaw throughthe pressure boundary.The following discussion on piping will address how Section III provides this protection, any variations that exist or that have existed between the piping rules and the design-by-analysis rules, and what items the analyst should be concerned with in satisfying Code requirements.Revisions in this chapter are based on ASME Boiler and Pres-sure Vessel Code 2010 Edition with 2011a Addenda, Section III, RULES FOR CONSTRUCTION OF NUCLEAR FACILITY COMPONENTS.Protection of the pressure boundary as discussed above is pro-vided for Class 1 components as a function of the stress cate-gories and stress limits applicable to those categories [12]. The stress categories that must be considered are as follows:(1)general primary membrane stress;(2)local primary membrane stress;(3)primary bending stress;(4)secondary stress; and(5)peak stress.Categories (1), (2) and (3) provide protection against cata-strophic failure. Categories (4) and (5) provide protection against fatigue failure [14]. Definitions of these terms are provided in NB-3213 of Section III; however, the main characteristics of these stresses are discussed in the following paragraphs.A primary stress is a stress developed by the imposed loading that is necessary to satisfy the laws of equilibrium between forces and moments external and internal to the component. A primary stress is not self-limiting. Once a primary stress exceeds the yield strength of the material through the entire thickness of a compo-nent, the prevention of failure is entirely dependent on the strain-hardening properties of the material.As noted in the preceding list, three categories of primary stress exist. The separation of membrane and bending components is attributed to the fact that a primary bending stress does not result in failure at the same level of allowable stress as does a primary mem-brane stress. The level of failure (exclusive of the strain-hardening properties of the material) is that the yield strength is exceeded through the entire wall thickness. The stress through the wall thick-ness varies for primary bending and is essentially constant for pri-mary membrane. Primary local membrane stress produced by mechanical loads has the characteristics of secondary stress in that it is self-limiting. When the stress exceeds yield, the load will be resisted by the remaining parts of the component since the localized area over which this stress is allowed to occur is controlled by Code rules. If not limited as a primary type stress, excessive distortion could occur that could be intolerable in some component locations. For piping, we find that there is no requirement to satisfy primary local membrane stress. The basic reason for this is that bend-ing is the major concern in piping systems and control of the general primary membrane stress due to pressure by use of a minimum thick-ness equation provides sufficient control. Certainly distortion of the pressure boundary is far more tolerable (in a geometric sense) in pip-ing than it is in a reactor pressure vessel, pump, or valve.A secondary stress is a stress developed by the self-constraint of adjacent material or of the structure. A secondary stress is self-limiting in that local yielding and minor distortions satisfy the conditions that cause the stress to occur. Secondary stressesS UBSECTION NB, NC,ND-3600 P IPINGJack R.Cole and Donald nders1 1Donald F. Landers was the original author who updated the Chapterfor the Second and Third Editions; for this fourth edition Jack R. Colerevised the chapter.8-2•Chapter 8normally occur as a result of geometric changes in a component configuration or thermal-type loads.A peak stress is the maximum stress that exists in the location of a component being evaluated. A peak stress causes no significant distortion and is a concern only with respect to continued cycling resulting in fatigue crack or brittle fracture. With the exception of some thermal stresses, peak stresses in piping are normally a result of a stress concentration factor.Thermal stresses are classified as secondary and peak stresses and are never considered primary stresses since they are self-limiting. Thermal stresses that produce distortion are placed in the secondary category and those that result from almost complete suppression of differential expansion and cause no significant distortion are classi-fied as peak stresses. The peak classification of some thermal stresses is worthy of discussion since one will find that the Class 1 rules of NB-3600 [13] treat these stresses differently than the design-by-analysis rules of NB-3200 [12]. To better understand this phenome-non, a little history is required. When Section III was first issued in 1965 the stress from a radial thermal gradient through the compo-nent wall was classified as a peak stress. This is explained in the Criteria Document [2] of Section III,a discussion of which follows.A special exception to the thermal rules is the case of the stress due to a radial gradient in a cylindrical shell. It is specifically stated that this stress may be considered a local thermal stress (i.e., peak stress). In reality, the linear portion of this gradient can cause defor-mation, but it was the opinion of the Special Committee that this exception could be safely made.Therefore, the design-by-analysis rules, when first published, considered the radial gradient as a peak stress. It must be noted that the Section III vesssel rules had a special stress limit requirement to prevent thermal stress ratchet of a shell subjected to thermal stress cycling in the presence of a static mechanical load. These rules provide a limit on the linear and parabolic variation of temperature through the wall. During the develop-ment of ANSI B31.7, “Nuclear Power Piping” [3], their Design Task Group decided to place the linear portion of the radial gra-dient into the secondary stress category rather than the peakstress category. It was recognized that this approach did notagree with the existing Section III requirements for vessels, which placed the stress resulting from this loading into the peak stress category. However, inclusion of the radial gradient stress in the secondary category in B31.7 eliminated the need to be overly concerned with thermal stress ratchet. When the rules for Class 1 piping in B31.7 were adopted into Section III in 1971, the vessel committee agreed, although grudgingly, to include the radial gradient in the secondary stress category. Inclusion of the radial gradient in the secondary category resulted in a number of cases where the limit on primary plus secondary stress intensity was exceeded, which resulted in the need to develop a simplified approach to elastic–plastic analysis. Again, B31.7 had developed a technique for ad-dressing this concern because of the need resulting from the decision to include the radial gradient in the secondary stress cate-gory [5]. When Section III adopted the piping rules of B31.7, they adopted a different approach to simplified elastic-plastic analysis [15]. The Section III approach is, of course, applicable to all com-ponents and the B31.7 technique was abandoned. The effect on industry of including the radial gradient as a secondary stress in piping will be discussed when the details of the applicable equa-tions in NB-3600 are addressed.Two other issues of importance are the discussion related to envi-ronmental fatigue effects and the development of a new correction factor for the simplified elastic plastic approach. Code Cases N-792 and N-761 which address methods of evaluation for the effect of reactor coolant environment on the wetted surface of the pressure boundary were recently published. Case N-792 provides a method for adjusting partial usage factors with an environmental penalty, Fen. Code Case N-761 provides a series of environmental fatigue curves that may be used to determine an adjusted environmental usage factor. Code Case N-779, titled Alternative Rules for Sim-plified Elastic-Plastic Analysis Class 1, Section III Division 1 provides adjustments to Ke for thermal stress terms in the alternating stress equations for piping. These cases will be discussed further below. Another very important development is the approval of a joint Section Ill-Section XI Code Case, N-755 related to the use of Polyethylene piping for buried application. This Code Case is dis-cussed further in 8.3.5.8.2NUCLEAR CLASS 1, NB-3600With the acceptance of ANSI B31.7 and the adoption of piping design rules in Section III, the piping design industry was sub-jected to dramatic changes that resulted in a significant increase in analytical manpower, a proliferation of computer analysis, and the loss of the piping designer. Despite the fact that the rules have been in existence since the publication of B31.7 in 1969, there still remains some confusion regarding the proper application of these rules. It is hoped that this discussion will help to alleviate that confusion.Based on a review of NB-3600 and NB-3200, the two sections appear to be completely different. In NB-3200, little guidance is provided in the areas of load or stress calculation, but consider-able discussion and guidance is given concerning stress character-istics, categories, allowables, and criteria. In NB-3600 there is basically no discussion of these issues, but equations are provided to calculate the required stresses to be compared with allowables. As the requirements of NB-3600 are discussed, the important areas will be compared to those of NB-3200. We will see that for primary stress protection, NB-3200 and NB-3600 are quite differ-ent, but in the secondary and peak stress areas, including fatigue, the basis for the rules is essentially the same although the analyti-cal approach is quite different.In developing rules for piping design in ANSI B31.7, the com-mittee took advantage of the existence of industrywide standards for pipe and pipe fittings [4], [6], [21] and [22]. These standards provide a reasonable control on geometry and therefore it is assumed that a specific fitting will respond to an applied load in a known manner. For example, a 4 in. schedule 40 elbow purchased to the same standard will deform in the same manner when sub-jected to an in-plane bending moment, whether it is in a nuclear or a fossil plant. This led to the use of stress indices (B, C, and K factors) that allow the engineer to calculate the stress in a straight piece of pipe of the same diameter and wall thickness as the fit-ting and multiply that value times an index to calculate the stress in the fitting for the intended protection (primary, secondary, or peak). The use of standard fittings that provide control on geome-try and allow the use of stress intensification factors (i values) was first introduced in B31 to calculate thermal expansion stresses. Significant discussion of stress indices and stress intensification factors is provided in Chapter 38.8.2.1Satisfaction of Primary Stressesfor Design ConditionsThe NB-3600 approach to the satisfaction of primary stresses relies on both design-by-rule and design-by-analysis methods. The first task is to determine wall thickness. The equations in NB-3600COMPANION GUIDE TO THE ASME BOILER & PRESSURE VESSEL CODE •8-3for required wall thickness are no different than they were in the past in the ANSI B31 Codes [8] and [21]. One issue that is proven to be critical in the industry is the failure to consider service environment and flow conditions when determining wall thickness. The required information for making this determination must be in the Design Specification and should include proper material selection to pre-clude attack or at least sufficient warning to the user. The code pro-vides guidance on types of environmental effects [31]. The piping analyst cannot be expected to make these decisions; his/her responsi-bility is to satisfy the Code by using the Design Specification require-ments. An inadequate Design Specification used by the analyst to sat-isfy Code requirements does not assure pressure boundary integrity.(a) Primary Membrane (NB-3640)The equations provided in NB-3641.1 for determining the minimum wall thickness of piping are as follows:(8.1)(8.2)Definitions of the equation terms are found in NB-3641.1.Note that the term A is partially defined as an additional thickness to provide for material removed in threading, corrosion, or erosion allowance. It is this A factor that requires sufficient input and guid-ance from others for the analyst to provide an appropriate value to increase the wall thickness to preclude failure. With the exception of rolled and welded pipe, once t m is determined the next higher schedule size is usually used. It must be recognized that the stan-dards for pipe allow a tolerance on the wall thickness. Depending on the standard this tolerance could be as large as 12 1͞2% resulting in a reduced wall thickness of that value. It must be assumed by the designer that the manufacturer will take advantage of any toler-ance allowed due to the financial incentive of the material saved.Determining minimum thickness satisfies a portion of the pri-mary stress requirements—that is, primary membrane stress. For standard fittings purchased and used in accordance with the requirements of NB-3691 no minimum thickness analysis is required. The basis for this is that fittings that satisfy the ANSI standards [22], [6], [23] are considered acceptable because their pressure-temperature ratings are based on burst tests, thereby assuring the fitting will withstand the design pressure. The engi-neer must assure that short radius elbows manufactured in accor-dance with ANSI B16.28 [4] have a minimum thickness in the crotch region 20% greater than required by the pipe schedule;(NB-3642.2). This is required to maintain the pressure stresses in the crotch region at an acceptable level. The pressure stress in a toroidal shape, such as an elbow, varies linearly across the toroid,being minimum at the extrados and maximum at the intrados.This variation and maximum value is a function of the toroidal radius-that is, a short radius elbow has higher stresses due to the same pressure in the intrados than does a long radius elbow of the same diameter and wall thickness. There is some question whether this is required (the author does not agree with the requirement) since the maximum stress is so localized; however,because the Committee has made this a requirement it must be satisfied. The designer should note that satisfying this 20%increase in wall thickness may result in a higher schedule elbow than the adjoining pipe which is going to result in secondary stresses which would not exist if the same schedules were used.For pipe bends, the wall thickness after bending must satisfy the minimum thickness requirements. Table NB-3642.1(b)-1 providest m =P d +2A (S m +P y )2(S m + Py -P )t m =PD o2(S m + Py )+ Aguidance on the wall thickness to be used prior to bending. Note that bending pipe results in a thinning of the wall on the extrados.It is important not to use excessive wall thickness since crimping of the metal can occur in the intrados, resulting in the potential for stress concentrations to exist.For intersections, a set of different rules applies. Tees manufac-tured in accordance with an ANSI or MSS standard listed in Table NCA-7100-1 are acceptable for satisfying primary membrane stress requirements. Branch connections not manufactured to a standard listed in Table NCA-7100-1, and those that are fabri-cated, must satisfy the reinforcement requirements of NB-3643.3.These reinforcement rules require that the area of metal removed for the branch connection must be available in a limited distribu-tion area around the opening—that is, the portion of pipe mate-rial that has been removed that carried membrane stress must be replaced in close proximity to the area removed. These rules for intersections and other standard fittings have been in place for many years and are based on the ASME B31.1 approach to pro-tection against burst-type failure.The fact that minimum thickness and the use of standard (pressure-temperature rated) fittings both satisfy the primary mem-brane requirements is a departure from the design-by-analysis requirements. This should make the user aware that piping Codes have been based historically on pressure protection and the use of pressure-temperature consideration has always been very important and successful. In design by analysis, the average stress intensity in the component wall must be calculated due to pressure and other design mechanical loads, and this stress intensity is compared to the allowable stress value, S m . For NB-3600, the average stress in the pipe wall due to design mechanical loads is not considered a pri-mary membrane stress. The stress resulting from these external loads is considered in the next step of primary stress protection.The following paragraphs address the resolution of primary stresses for Design Conditions only; primary stress requirements for Levels A, B, C, and D operating conditions are discussed later.(b) Primary Membrane Plus Bending (NB-3652)This title is a bit misleading since the approach in piping is again quite different than that for design by analysis. In piping, Equation (9) is provi-ded to satisfy what is defined in NB-3200 as primary membrane plus primary bending stress intensity. This equation is really a limit load-based equation since it provides the maximum longitu-dinal stress, rather than a maximum shear stress, in a piping com-ponent. For example, in a thin shell cylinder, the stresses due to pressure are shown in the equations that follow.For axial stress:(8.3)For hoop stress:(8.4)For radial stress:(8.5)The maximum shear stress is or . In Equation (9), the pressure term is:(8.6)B 1PD o 2t(PD o /2t )+P s H -s R s R =-Ps H =PD o 2ts x =PD o 4t8-4•Chapter 8On the surface this appears to represent the hoop stress in a cylinder. However, the factor is important; for a straight piece of pipe (a cylinder), . Therefore, the pressure term in Equation (9) for a straight piece of pipe represents the axial stress due to pressure, .Equation (9) is provided in NB-3652 as follows:(8.7)whereϭprimary stress indices for the specific product underinvestigation (NB-3680)P ϭdesign pressure, psiD o ϭoutside diameter of pipe, in. (NB-3683)t ϭnominal wall thickness of product, in. (NB-3683)I ϭmoment of inertia, in.4(NB-3683)M i ϭresultant moment due to a combination of designmechanical loads, in. lb. All design mechanical loads and combinations thereof shall be provided in the Design Specification. In the combination of loads, all directional moment components in the same direction shall be combined before determining the resultant moment—that is, resultant moments from different load sets shall not be used in calculating the moment (M i ). If the method of analysis for earthquake or other dynamic loads is such that only magnitudes without relative algebraic signs are obtained, the most conser-vative combination shall be assumed.S m ϭallowable design stress intensity value, psi.It should be noted that Eq. (9) allows the use of the nominal wall thickness of the piping product so that the manufacturing tole-rances need not be considered. In fact NB-3683.1 notes that the nominal wall thickness shall be used for evaluating Eq. (9) through (14) and for calculating stress indices. For buttweld fittings the nominal wall thickness of the equivalent pipe shall be used. That is, for a schedule 40 pipe cap the thickness used shall be that for a schedule 40 pipe with no tolerance applied. Note the discussion after Eq. (11) which requires the consideration of the allowance.The pressure term was discussed previously. The moment term,without the stress index B 2, is merely the axial bending stress in a straight cylinder due to an external moment. Therefore, Equation (9)provides the axial stress in a piping component. The limit on this stress is based on limit load design theory and assumes that the material is elastic-perfectly plastic with no strain ing this assumption, the stress limit was developed by provid-ing a margin on the actual limit load stress curve for combined tension and bending on a rectangular section. This is shown in Fig. 8.1. Quite often, the point is made that 1.5 is the “shape fac-tor” for a particular section and should not be used for hollow cir-cular sections. This point is usually made in reference to the allowable stress of 1.5S m for primary bending for Class 1 compo-nents. It is important to recognize that the 1.5 factor used with S m is not an attempt to provide a “shape factor”; rather, it is a factor that, when taken with the allowable stress (S m ), provides a margin on the theoretical limit load for any elastic–perfectly plastic material. For ferritic materials, S m can never be higher than S y ;therefore, 1.5S m results in an allowable stress for primary mem-brane plus primary bending that is never higher than S y on the outside wall of the pipe. Figure 8.1 indicates that the theoretical limit stress varies from 1.5S y with no membrane stress present to 1.0S y with only membrane stress present. It should be noted that23B 1, B 2B 1PD o 2t+B 2D o2I M i… 1.5S m PD o /4t B 1=0.5B 1the theoretical limit stress peaks at approximately 1.65 with a combination of bending and membrane stress when the mem-brane stress is approximately S y . The theoretical limit of 1.65should not be used for design purposes since it would negate the margins of safety.In reality, Figure 8.1 does not represent the margin between Code allowable and theoretical limit load for a straight cylinder that has a shape factor of about 1.33 instead of 1.5. However, it should be recognized that for elbows, the addition of membrane stresses due to pressure increases the capacity of the elbow to carry a bending moment. This is recognized in the winter 1981addenda to NB-3600 of Section III that provided an equation to determine B 1, which considered the effect of pressure on collapse of an elbow. This effect varies from zero to a value equal to that for a straight pipe. This is based on the fact that an elbow subjected to an in-plane moment ovalizes. That ovalization is resisted by the internal pressure. This resistance is a function of the magnitude of the pressure and the thickness of the elbow. Another point of interest is that the maximum stress in an elbow due to an in-plane moment occurs at what could be called the neutral axis and it is not a beam bending type stress as it is for a straight pipe. This stress results from the ovalization that occurs and is positive on the outer surface and negative on the inner surface, essentially resulting in zero membrane stress. Normally, in most piping systems, the elbows are considered the weak link with respect to collapse resulting from external mechanical loads.(c) Moment Calculation The fact that the stress calculated in Equation (9) is a longitudinal stress and the allowable is based on limit load restrictions assuming elastic-perfectly plastic material with no strain hardening is one part of the picture; the other part is calculation of loads. The value of P is given as the design pressure13FIG.8.1LIMIT LOAD CURVECOMPANION GUIDE TO THE ASME BOILER & PRESSURE VESSEL CODE •8-5and is provided in the Design Specification. The moment M i must be calculated and considers those design mechanical loading con-ditions provided in the Design Specification. It must be noted that NCA-2142.1 [1] is quite specific in indicating that Design Loadings are those that exist under the most severe Level A loading conditions. Based on this, design pressure, design tem-perature, and design mechanical loads may not be the maxi-mum values that the piping system is subjected to since higher conditions may exist for other Service Level loadings. This is unique to Section III design, and exists because a number of postulated events must be considered to provide the necessary protection associated with nuclear power generation. That is, con-ditions other than those associated with the operation of the plant must be considered. Some of these conditions are earthquakes of such magnitude that they have a low probability of occurrence, as well as large pipe rupture and small pipe breaks.Load calculation, which is of major importance, is the item that is not addressed in NB-3600 just as it is not addressed in NB-3200. One can find great detail and limitations on the calcula-tion of stress indices [18] and [19], including those to be used for detailed analysis (NB-3684), but little if any guidance on load cal-culation. Of course, the determination of the moment values to be used in the individual equations of NB-3650 is critical to the value of calculated stress, and some in the industry believe that it is more important than the stress indices.For example, conservative assumptions made with respect to thermal expansion or anchor point loads may not be conservative when considering dynamic loading. For thermal expansion, the assumption that anchors, equipment nozzles, and supports are rigid is a conservative one. In general, this is not the case for seismic loading where the most standard approach is to have a design where the fundamental frequency of the piping system is above the peak of the spectra. Flexible anchors, equipment nozzles, and sup-ports will result in a lower frequency than calculated with those items rigid, which could result in the actual design frequency being in the peak of the broadened spectra. In fact, the accurate calculation of piping moment loading is one of the most difficult analytical problems that exist in meeting Code compliance. There are many variables that have an effect on the actual moment value, some of which were mentioned previously. In many cases where the results of standard analytical techniques for determining thermal expansion loads were compared to experimental results, there have been large variations. In some cases, the analytical values, were greater than the experimental; in others, they were less. This is rather ironic when compared to the details provided in the Code with respect to calculating stress indices. Piping design history, including that in the nuclear power field, indicates that failures as a result of thermal expansion are highly extraordinary. Failures as referred to here are violation of the pressure boundary. The majority of failures in piping in nuclear plants have resulted from erosion, erosion-corrosion, or vibration. Note that the first two causes can be minimized by material selection, additional wall thickness beyond Code requirements, chemistry control, or other measures that essentially are outside the scope of the piping analyst. Although NB-3613.1, “Corrosion or Erosion,” states that the wall thickness of the piping shall be increased over that required by other design requirement and shall be consistent with the specified design life of the piping, the analyst/designer normally is not qual-ified to determine this. The Design Specification must provide the required information or require the use of a material that resists these phenomena. The third cause, vibration, usually results from poor design of such items as drains, strainers, and other small branch connections that were not supported properly. NB-3622.3requires that piping shall be arranged and supported so that vibra-tion will be minimized. It further requires that the designer shall be responsible, by design and by observation under start-up or initial service conditions, for ensuring that vibration is within acceptable levels. The designer should not depend only on startup testing to reveal piping vibration issues. When piping is attached to rotating equipment such as pumps, it is possible to anticipate the excitation frequencies that may be susceptible to steady state flow induced vibration. The pump 1X and vane passing frequencies could possi-bly excite the attached piping. [41] Effort should be made during the design stage to evaluate the piping frequencies that may be sub-jected to vibration excitation during operation. The piping model should be built to capture the frequencies of interest and should be evaluated in the design phase. This provides a better design such that changes during start up or the operational test phase are mini-mized. Methods for evaluation are available to guide the blending of design vibration analysis with operational testing. [42] Without planning in the design stage, there may be delays during startup testing while systems are modified to prevent undue vibration. There have been some cases of large distortion and a few failures due to water or steam hammer. These generally occurred due to the fact that the loading, or potential for the loading, was not defined in the design documents. Had they been, the piping designer could have prevented damage to the piping system.With respect to the moment term in Equation (9) for design conditions, the designer is dealing only with weight loading; the solution is not a concern, nor is it affected considerably by the stiffness assumptions discussed earlier. In reality, the designer will have located supports prior to satisfying Equation (9) by using a standard hanger spacing table, most of which are based on simply supported beams using an allowable stress on the order of 5000 psi or less. In locating supports, the designer should attempt to place them where vertical displacement is minimal so that their effect on thermal expansion will be slight. Doing so at this point in the design stage is a judgment call that relies on the experience of the designer. The thermal expansion analysis will verify that experience. A more detailed discussion of this issue and a proce-dure for design process is provided in Section 8.4.In the definition of M i there is discussion of earthquake loading and how the moment values generated as a result of that analysis must be handled. As discussed previously, for Design Conditions the designer should not find a requirement to analyze for a seis-mic event in the Design Specification. The discussion under the definition of M i is there to deal with protection associated with dynamic events that are classified as Level B, C, or D in the Design Specification.In summary, primary stress protection for Design Conditions in NB-3600 is as follows:(1)calculation of minimum wall thickness;(2)use of standard fittings;(3)area replacement rules for intersections;(4)20% increase in short radius wall thickness;(5)assurance that the extrados of bends satisfies minimum wallthickness; and(6)satisfaction of Equation (9).8.2.2Fatigue, Elastic Action Requirements(NB-3653 and NB-3654)The NB-3650 requirements for fatigue protection follow the design-by-analysis requirements of NB-3200 [9]. The major differ-ence is that NB-3650 defines how the stresses will be calculated. It。
ASME动力管道
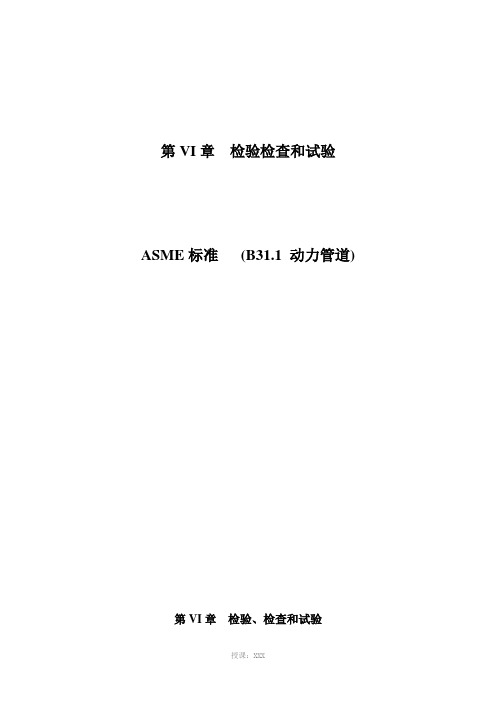
第VI章检验检查和试验ASME标准(B31.1 动力管道)第VI章检验、检查和试验136 检验和检查;136.1 检验136,1.1 概述本规范将“检查(examination)”和“检验(inspection)两术语加以区别。
检验是业主的责任,除去第136.2节所要求的检验外,它可以由业主的雇员或业主授权的某一机构来执行。
初始运行之前,应检验管道装置以保证其符合工程设计性能和本规范的材料、加工、装配、检查和试验等方面的要求。
136.1,2 符合性验证当要求使用ASME锅炉及压力容器规范第1卷规定的规范钢印标记时,应由授权检验师检验其是否符合本规范的要求。
应采用本规范的规则和ASME锅炉及压力容器规范第1卷附录A—300的质量控制体系的要求。
质量控制体系的要求见本规范附录J。
检验师的职责应按ASME锅炉及压力容器规范第1卷PG—90的规定的执行。
数据报告表的格式已附列在ASME锅炉及压力容器规范第1卷附录内,用于汇总必要的检查记录。
检验师本人应确认管道系按照本规范的有关要求进行制造的。
对焊缝进行无损检查,的人员应按雇主制定的认证人员大纲对每项检查方法进行评定和认证。
大纲,应按下列最低要求制订:(A)无损检查方法基本原理方面的说明;(B)在职培训无损检查人员使熟悉焊缝缺陷显示的形状和评判。
培训时间应确保能充分掌握所需要的检查知识;(C)无损检查人员应进行每年≥1次视力检查以确定其从事所要求检查的视觉能力;(D)一旦完成上述(A)和(B)要求后,无损检查人员应接受雇主的口头或书面考试及操作技能考试以确定其是否有资格从事所要求的检查和结果的评判工作;(E)凡已取证的无损检查人员如有≥1年没有从事规定的检查方法工作,应按上述(D)项要求进行重新考核,同时还得通过(C)项的视力检查。
若工艺或设备有实质性修改和变化,应对无损检查人员重新进行资格评定。
可以用下述标准作为替代上述大纲的另一种考评办法:SNT—TC—lA或CP—189和按AWS QCl进行焊缝目视检查人员资格评定。
动力管道标准
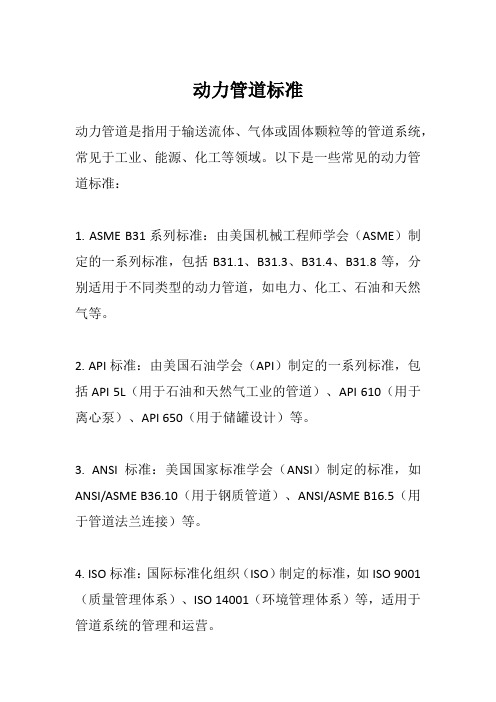
动力管道标准
动力管道是指用于输送流体、气体或固体颗粒等的管道系统,常见于工业、能源、化工等领域。
以下是一些常见的动力管道标准:
1. ASME B31系列标准:由美国机械工程师学会(ASME)制定的一系列标准,包括B31.1、B31.3、B31.4、B31.8等,分别适用于不同类型的动力管道,如电力、化工、石油和天然气等。
2. API标准:由美国石油学会(API)制定的一系列标准,包括API 5L(用于石油和天然气工业的管道)、API 610(用于离心泵)、API 650(用于储罐设计)等。
3. ANSI标准:美国国家标准学会(ANSI)制定的标准,如ANSI/ASME B36.10(用于钢质管道)、ANSI/ASME B16.5(用于管道法兰连接)等。
4. ISO标准:国际标准化组织(ISO)制定的标准,如ISO 9001(质量管理体系)、ISO 14001(环境管理体系)等,适用于管道系统的管理和运营。
5. GB标准:中国国家标准,如GB 50205(工业金属管道设计规范)、GB/T 12771(不锈钢焊接钢管)等。
这些标准涵盖了管道系统的设计、制造、安装、测试和运行等方面的要求,旨在确保管道的安全、可靠和符合法规标准。
具体适用的标准取决于管道所属行业、管道材料、输送介质等因素,建议根据具体需求选择相应的标准进行参考和遵循。
- 1、下载文档前请自行甄别文档内容的完整性,平台不提供额外的编辑、内容补充、找答案等附加服务。
- 2、"仅部分预览"的文档,不可在线预览部分如存在完整性等问题,可反馈申请退款(可完整预览的文档不适用该条件!)。
- 3、如文档侵犯您的权益,请联系客服反馈,我们会尽快为您处理(人工客服工作时间:9:00-18:30)。
第VI章检验检查和试验ASME标准(B31.1 动力管道)第VI章检验、检查和试验136 检验和检查;136.1 检验136,1.1 概述本规将“检查(examination)”和“检验(inspection)两术语加以区别。
检验是业主的责任,除去第136.2节所要求的检验外,它可以由业主的雇员或业主授权的某一机构来执行。
初始运行之前,应检验管道装置以保证其符合工程设计性能和本规的材料、加工、装配、检查和试验等方面的要求。
136.1,2 符合性验证当要求使用ASME锅炉及压力容器规第1卷规定的规钢印标记时,应由授权检验师检验其是否符合本规的要求。
应采用本规的规则和ASME锅炉及压力容器规第1卷附录A—300的质量控制体系的要求。
质量控制体系的要求见本规附录J。
检验师的职责应按ASME锅炉及压力容器规第1卷PG—90的规定的执行。
数据报告表的格式已附列在ASME锅炉及压力容器规第1卷附录,用于汇总必要的检查记录。
检验师本人应确认管道系按照本规的有关要求进行制造的。
对焊缝进行无损检查,的人员应按雇主制定的认证人员大纲对每项检查方法进行评定和认证。
大纲,应按下列最低要求制订:(A)无损检查方法基本原理方面的说明;(B)在职培训无损检查人员使熟悉焊缝缺陷显示的形状和评判。
培训时间应确保能充分掌握所需要的检查知识;(C)无损检查人员应进行每年≥1次视力检查以确定其从事所要求检查的视觉能力;(D)一旦完成上述(A)和(B)要求后,无损检查人员应接受雇主的口头或书面考试及操作技能考试以确定其是否有资格从事所要求的检查和结果的评判工作;(E)凡已取证的无损检查人员如有≥1年没有从事规定的检查方法工作,应按上述(D)项要求进行重新考核,同时还得通过(C)项的视力检查。
若工艺或设备有实质性修改和变化,应对无损检查人员重新进行资格评定。
可以用下述标准作为替代上述大纲的另一种考评办法:SNT—TC—lA或CP—189和按AWS QCl进行焊缝目视检查人员资格评定。
136.1.3 检验师的权利检验师应能随意出入正在从事管道制作的现场,包括管道的制造、加工、装配、安装和试验等场所。
检验师应有权查阅在第136.1.1节检验要求中涉及的所有合格证件和档案记录包括焊工或焊接操作工评定及WPS评定的合格证明资料,136.1.4 业主检验师的资格评定(A)业主检验师应由业主指定并应是业主的雇员、工程或科学组织的雇员或作为业主代理的公认保险或检验公司的雇员。
业主雇员应既不代表管道制造商、制作者或安装者也不是其雇员,除非业主也是制造商、制作者或安装者。
(B)业主检验师应有≥10年在设计、制造、安装、制作或检验动力管道的经验。
由工程技术鉴定委员会认可的满意地完成工程等级的每1年相当于1年的经验,总共可达5年。
(C)在委派检验操作中,业主对指定人员所指派的检验功能经过评定负责。
136.2 授权检验师对锅炉围管道的检验和评定136.2.1 按第100.1.2(A)节确定需要进行检查和打钢印的管道应在制造过程中和完工后以及在授权检验师选定的任何工作阶段进行检查。
具体要求见ASMEE锅炉及压力容器规第1卷中PG-104~PG-113,各制造制作和装配单位要安排支持授权检验师的业务活动.136.2.1.1 本卷要求的检验应由ASME认可的授滇检验机构雇用的检验师完成。
136.2.2 若要求用打钢印和用数据报告证明合格,都应按ASME 锅炉及压力容器规第1卷定PG—104、PG—105、PG—109、PG—110、PG—111和PG—112的规定执行。
136.3 检查136.3.1 概述“检查“表示由制造厂、加工厂、安装单位或业主授权的某一机构来完成的职能,其方法包括肉眼观察和无损检查如射线照相、超声波、涡流、液体渗透和磁粉检验等探伤方法。
检查程度和验收标准超出本规要求的,应由制造厂、制作或安装单位与业主事先协定。
136,3.2 NDE人员资格评定对焊缝进行无损检查的人员应按雇主制定的认证人员大纲对每项检查方法进行评定和认证。
大钢应按下列最低要求制订:(A)无损检查方法基本原理方面的说明;(B)在职培训无损检查人员使熟悉焊缝缺陷显示的形状和评判。
培训时间应确保能充分掌握所需要的检查知识;(C)无损检查人员应进行每年≥1次视力检查以确定其从事所要求检查的视觉能力;(D)一旦完成上述(A)和(B)要求后,无损检查人员应接受雇主的口头或书面考试及操作技能考试以确定其是否有资格从事所要求的检查和结果的评判工作;(E)凡已取证的无损检查人员如有≥1年没有从事规定的检查方法工作,应按上述(D)项要求进行重新考核,同时还得通过(C)项的视力检查。
若工艺或设备有实质性修改和变化,应对无损检查人员重新进行资格评定。
可以用下述标准作为替代上述大纲的另一种考评办法:SNT—TC—lA或CP—189和按AWSQCl进行焊缝目视检查人员资格评定。
136.4 焊缝的检查方法136。
4.1无损检查无损检查应按本章要求进行。
压力焊缝和承压组件焊缝的强制性检查的类型和围规定在表136.4中。
表136.4以外的焊缝,只要求进行目视检查。
要求进行无损检查的焊缝应符合适用的对显示的验收标准,如第136.4,2~136.4,6节所示。
作为指南、各种检查方法的检测能力见表136.4.1按本规或工程设计不要求进行检查(即RT、UT、PT或MT)的焊缝如能满足第136.4.2节的检查要求和第137节规定的压力试验要求,则应判为合格。
136.4.2 目视检查当制作和安装管道组件时,若有必要验证符合设计和WPS的要求,应进行按第100.2节定义的目视检查。
此外,目视检查应被用来验证管道和管道元件上完工的焊缝符合下列(A)规定的验收标准或者符合管道组件供货时材料标准中规定的有关缺陷限制值。
(A)验收标准下列显示作不合格论处。
(A.1)裂缝——外表面;(A.2)咬边深度>1/32in(1.0mm);(A.3)焊缝佘高大于表127.4.2的规定值;(A.4)表面未熔合;(A.5)未焊透(仅适用于可以进入表面工作的情况);(A.6)任何其它线性显示长度>3/l6in(5.0mm);(A.7)圆形显示尺寸>3/16in(5.0mm)的表面缩孔或≥4个的圆形显示,其分散间距或边缘间距在任何方向<1/16in(2.0mm)。
圆形显示是指圆或长度小于3倍宽度的椭圆。
136.4.3 磁粉检查当本章(见表136.4)有要求时,磁粉检查应按ASME锅炉及压力容器规第v卷第7章的方法进行。
(A)缺陷显示的评定(A.1)在表面的机械不连续性现象是由于检查所使用的耦合介质残留来显示的。
但是并非所有的显示图像都是缺陷,如某些冶金不连续性和导磁率的变化可能产生类似的、与检测不合格缺陷无关的显示图像。
(A.2)凡认为可能是非相关的显示均应进行复检以确定是否有真缺陷。
复检前可以进行表面修整。
凡掩盖缺陷显示的非相关显示是不合格的。
表136.4 受压焊缝或承压组件焊缝最低限度强制性无损检查要求说明:(A)所有焊缝,除了进行规定的特定无损检查外还必须进行目视检查,(B)NPS--公称管道规格。
(C)PT——射线照相检查,UT——超声波检查,MT--磁粉检查,PT--液体渗透检查,VT——目视检查。
(D)有压力级组件的无损检查参阅表126.1所列标准或制造技术条件。
{E)无损检查验收标准如下:MT见第136.4.3节,PT见第136.4.4节,VT见第136.4.2节,RT见第136.4.5节,UT 见第136.4.6节;注:(1)对接焊缝厚度系指端部开坡口后,相对接两瑞的较厚一侧的厚度。
(2)当按第136.4.5节执行时,可以用RT宋代替或MT或PT,(3)支管焊缝的RT或UT应在焊非整体补强材料前进行。
(4)允许以表面检查(PT或MT)来代替上述所要求的对于焊制支管接头焊缝的体积检查(RT或UT);在使用时应在1/2焊缝厚度以或每1/2in(12.5mm)焊缝厚度和所有可抵及的最终焊缝表面上进行检查。
(5)当非压力级零件的永久性附件上角焊缝厚度≤1/4 in(6mm)时,无须按上表要求进行PT或MT。
表136.4.1 各种检查方法所能显示的焊缝缺陷注:(1)用于外表面可抵及的检查和/或当表面可抵及时也包括表面。
(2)当其不连续性开口抵及表面时才可检测。
(3)仅适用于易抵及的表面。
(A.3)相关显示系指那些由不能接受的机械不连续性产生的缺陷显示,线性显示系指其长度>3倍宽度的缺陷显示。
圆形显示系指圆形或长度<3倍宽度的椭圆形显示。
(A.4)不连续性显示很可能比实际的不连续性缺陷大,然而合格或判废的依据是缺陷显示的尺寸而不是不连续性的实际尺寸。
(B)验收标准下列相关显示作为不合格论处:B.1) 任何裂纹或线性显示;B.2) 圆形缺陷显示尺寸>3/16in(5.0mm);B.3) 圆形缺陷显示>4个,呈直线分布其边缘相隔间距≤1/16。
in(2.0mm)者;(B.4) 在任意6in2(3870mm2)面积上,有≥10个圆形显示,该面积的最大尺寸从相对评定显示最不利的部位取<6 in(150mm)。
136.4.4 液体渗透检查当本章(见表136.4)有要求时,液体渗透检查应按ASME锅炉及压力容器规第v卷第6章的方法进行。
(A) 缺陷显示的评定(A.1) 表面机械不连续性是由渗出的渗透剂来显示的;然而,局部的表面缺陷如由机械加工划痕或表面状况也可能导致类似的缺陷显示,与检测不合格缺陷无关。
(A.2) 凡认为可能是非相关的显示均应被视为缺陷并进行复检以确定其是否是真缺陷。
复检前可以进行表面修整。
凡掩盖缺陷显示的非相关显示和大面积色素淀积是不合格的。
(A.3) 相关显示系指那些由不能接受的机械不连续性产生的缺陷显示。
线性显示系指长度>3倍宽度的缺陷显示。
圆形缺陷显示系指圆形或长度<3倍宽度的椭圆形显示。
(A.4) 不连续性缺陷显示很可能比实际的不连续性缺陷大,然而合格或判废的依据是缺陷显示的尺寸、而不是不连续性缺陷的实际尺寸作为合格或判废的依据的。
(B) 验收标准主要尺寸>1/16in(2.0mm)的缺陷显示,应考虑作为相关的缺陷显示,下列缺陷的相关显示作为不合格论处:(B.1) 任何裂纹或线性显示,(B.2) 圆形缺陷显示>3/16in(5.0 rn rn);(B.3) 圆形缺陷显示≥4个呈直线分布,其边缘相隔间距≤1/16in(2.0mm);(B.4) 在任意6in2 (3870 rnrn2)面积上,有≥10个圆形显示,该面积的最大尺寸从相对评定显示最不利的部位取<6 in(150mm)。
136.4.5 射线照相检查当本章(见表136.4)有要求时,射线照相检查应按ASME锅炉及压力容器规第V卷第2章进行,除非几何不清晰度超过0.07in(2,0mm)按T—285节要求仅用作指南、不是作为射线照相的判废用。