LiMn2O4 cathode doped with excess lithium and synthesized by co-precipitation for Li-ion batteries
纳米LiMn2O4的制备
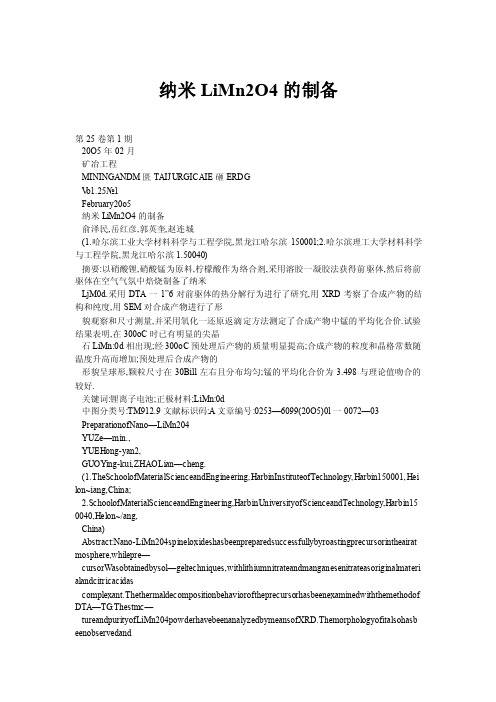
纳米LiMn2O4的制备第25卷第1期20O5年02月矿冶工程MININGANDM匮TAIJURGICAIE砸ERDGV o1.25№1February20o5纳米LiMn2O4的制备俞泽民,岳红彦,郭英奎,赵连城(1.哈尔滨工业大学材料科学与工程学院,黑龙江哈尔滨150001;2.哈尔滨理工大学材料科学与工程学院,黑龙江哈尔滨1.50040)摘要:以硝酸锂,硝酸锰为原料,柠檬酸作为络合剂,采用溶胶一凝胶法获得前驱体,然后将前驱体在空气气氛中焙烧制备了纳米LjM0d.采用DTA一1”6对前驱体的热分解行为进行了研究,用XRD考察了合成产物的结构和纯度,用SEM对合成产物进行了形貌观察和尺寸测量,并采用氧化一还原返滴定方法测定了合成产物中锰的平均化合价.试验结果表明,在300oC时已有明显的尖晶石LiMn:0d相出现;经300oC预处理后产物的质量明显提高;合成产物的粒度和晶格常数随温度升高而增加;预处理后合成产物的形貌呈球形,颗粒尺寸在30Bill左右且分布均匀;锰的平均化合价为3.498与理论值吻合的较好.关键词:锂离子电池;正极材料;LiMn:0d中图分类号:TM912.9文献标识码:A文章编号:0253—6099(20O5)0l一0072—03 PreparationofNano—LiMn204YUZe—min.,YUEHong-yan2,GUOYing-kui,ZHAOLian—cheng.(1.TheSchoolofMaterialScienceandEngineering,HarbinInstituteofTechnology,Harbin150001,Hei lon~iang,China;2.SchoolofMaterialScienceandEngineering,HarbinUniversityofScienceandTechnology,Harbin15 0040,Helon~/ang,China)Abstract:Nano-LiMn204spineloxideshasbeenpreparedsuccessfullybyroastingprecursorintheairat mosphere,whilepre—cursorWasobtainedbysol—geltechniques,withlithiumnitrateandmanganesenitrateasoriginalmateri alandcitricacidascomplexant.Thethermaldecompositionbehavioroftheprecursorhasbeenexaminedwiththemethodof DTA—TG.Thestmc—tureandpurityofLiMn204powderhavebeenanalyzedbymeansofXRD.Themorphologyofitalsohasb eenobservedandgrainsizehasbeenmeasuredbySEM.ThemeanvalencyofmanganeseinLiMn204hasbeendetermined byredoxtitrationmethod.Resultsshowthatthere’resomeobviousspinelstructureofLiMn204at300oC.Andthequalityo fLiMn204Canberemarkablyimprovedafterbeingpretreatedat300oC.Thegrainsizeandlatticeconstanttendtoincrease withtheincreaseoftemperature.Afterpretreatment,LiMn204isinthefonnofsphericit,distributingevenlywiththeaverage grainsizeof30Bin.Themeanvalencyofmanganeseis3.498,whichisveryclosetothetheoreticalvalue.Keywords:lithium—ionbattery;cathodematerial;LiMn204目前,可用作锂离子电池正极材料的主要有层状结构的LiCoO2,LiNiO2和尖晶石结构的LiMn204¨』.由于LiCo02的制备工艺简单,因而对LiCo02的研究最为成熟,已商品化.但LiCoO2存在价格昂贵,污染大,不耐过充和过放电的缺点.而LiNi02的制备条件控制非常苛刻,在制备过程中条件控制不同,很容易形成非化学计量的化合物,从而影响LiNi02的比容量和其它一些电化学性能.与以上2种正极材料相比,LiMn204具有的资源丰富,无毒性,价格便宜等优点,被认为是一种最具吸引力的正极材料H.但是,容量较低和循环寿命短尤其是高温性能差是困扰LiMn:04商品化应用的主要障碍j.合成方法和焙烧温度对LiMn~04电极的电化学性能有很大的影响j.LiMn~04的合成一般分为高温固相法和低温液相法.高温固相法合成温度高,时间长且所得产物粒度较大,尺寸不均匀,电化学性能较差.一般认为电极材料应具有单相,高纯,均一的颗粒和较窄的尺寸分布以及大的比表面积,有利于提高LiMn:04的容量L7』.为此本文拟采用低温液相法制备纳米LiMn~04,并对其制备工艺,物理性质进行详细的研究.①收稿日期:2OO4-O8.18基金项目:黑龙江十五攻关项目(编号:GC01A2201)作者简介:俞泽民(1961一),男,浙江人,博士研究生,副教授,研究方向为电子材料/材料物理与化学.第1期俞泽民等:纳米uMI】2o4的制备731LiMnO4的制备及测试方法1.1尖晶石型LIMn2o4的制备以硝酸锂和硝酸锰为原料,柠檬酸作络合剂,采用柠檬酸络合法制备了尖晶石型LiMn204(其中预处理时间和焙烧时间均为10h).工艺流程见图1所示.图1工艺流程1.2测试方法采用ZRY一2P差热.热重分析仪研究柠檬酸络合所得的干凝胶前驱体的热分解行为,以10~C/min的加热速率在空气气氛条件下进行加热,温度范围为室温~800oC.运用日本理学电机D/max—rB型x射线衍射仪进行物相和结构分析.通过氧化一还原返滴定法分析合成产物中锰元素的平均化合价.用PhilipsFEI Sirion一200扫描电镜观察样品形貌并测定颗粒大小, 工作电压是20kV.2结果和讨论2.1前驱体的热行为分析柠檬酸络合前驱体干凝胶在加热过程中的DTA—TG分析结果如图2所示.温度/XZ图2前驱体干凝胶差热一热重曲线从DTA曲线可以看出,柠檬酸络合法获得的前驱体在加热过程中有2个吸热峰和一个放热峰产生.在130cc的吸热峰与水蒸气的挥发有关,在210220cc的吸热峰是由焙烧材料的熔化产生的.在270~300o【=产生一个较大的放热峰,由TG曲线和图3可知,该放热峰是由于前驱体的分解及LiMn2o4的开始形成产生的.由TG曲线可以看出,180oC以前质量损失较小,损失量大约为3%,180~300oC质量损失迅速增大,损失量大约为70%,300~400oC质量损失显着减小,400oC以上质量无变化,表明在300oC以上凝胶分解过程基本完成.可见,LiMn:o4的合成温度应在300o【=以上,其反应的化学方程式为:taNO~(aq)+2Mn(N()3)2(aq)+3C6o7(aq)+11o2(g)—一LiMn2o4(s)+18CO2(g)十+12H2O(g)十+4NO(g)十+NO2(g)十2.2焙烧温度对合成产物的影响图3为干凝胶在不同温度(300~750oC)焙烧时的x射线衍射图.由图3可见,在300oC焙烧的产物虽然基线不太平整,背底相对较大但已具有很明显的尖晶石骨架特征峰,说明在300oC合成的LiMn2o4具有较完整的尖晶石结构.随着焙烧温度的升高,基底越来越平,而且半高宽变小,峰形渐尖锐,但产物中杂质相含量较多.在不同温度焙烧时的杂质种类及杂质含量见表1.由表1可知,当直接焙烧温度为750o【=时, 合成产物中杂质最多(约34%的Mn2),而经过300o【=预处理,750oC焙烧的产物杂质量最少(约3%的MnO,).产物的纯度对电池的电化学性能影响很大,因此可以认为经300oC预处理,750oC焙烧是合成LiMn2o4的最佳工艺条件.20/(.)图3不同温度焙烧制得LjMn2o4的XRD图谱74矿冶工程第25卷表1不同合成温度下焙烧产生的杂质种类及含量1)经300℃预处理.基于(111),(311),(400),(511)和(44O)晶面的X射线衍射峰,根据干涉晶面间距和干涉晶面指数的关系, 采用最-’bZ.乘法得到不同合成温度下产物的品格常数, 所得结果见表2.由表2可知,随着温度的升高,产物的品格常数大体呈增加趋势,但经300℃预处理,750℃焙烧的产物品胞常数较直接在750℃焙烧的小.表2不同合成温度下LiMo4的晶格常数和颗粒尺寸1)经300℃预处理.根据(111),(311),(222),(400)和(331)晶面的x射线衍射峰计算的颗粒尺寸(见表2)可以看出,随着温度的升高,产物的颗粒尺寸增加,而经300℃预处理,750℃焙烧的产物,其颗粒尺寸较750℃直接焙烧的小,这可能与形核率增大有关.2.3预处理对合成产物的影响由表1可以看出,直接在750℃焙烧的LiMn204的纯度为65%;经过300℃预烧,750℃焙烧的LiMn204的纯度提高到97%,说明预处理可显着提高LiMn204的纯度.如前所述,这是由于在300℃预处理时前驱体分解基本完成,而余下的Li,Mn和空气中的氧直接合成为LiMn204.而不经预处理直接在750℃焙烧时,前驱体中的有机物发生碳化,使一些Li和Mn 被碳隔离,在空气中被氧化成Mn2O.2.4扫描电镜(SEM)分析图4是样品在300℃预处理,750℃焙烧的扫描电镜照片.由图4(a)可以看出,LiMn204颗粒呈球形,有团聚现象,颗粒尺寸分布较均匀.图4(b)是分散后颗粒的扫描电镜照片,其形貌呈球形,颗粒大小约为30 nIn且尺寸大小均匀,颗粒尺寸与XRD—LB计算的结果基本吻合.可见,本试验所制备的LiMn204具有颗粒尺寸小,尺寸分布均匀且球形度良好的特点,是理想的正极材料.图4300oC预处理750oC焙烧样品(a)团聚的;(b)分散的颗粒2.5合成产物中锰的平均化合价的分析在完整的尖晶石结构LiMn204中,锰的平均价态为+3.5价.本试验采用氧化.还原返滴定法测定了合成产物中锰元素的平均价态.试验测得在300~C预处理,750℃焙烧产物中锰的平均化合价为3.498,与理论值相吻合.3结论1)柠檬酸络合前驱体干凝胶在270~300℃发生分解,在300℃已经生成尖晶石结构LiMn204.2)随着焙烧温度的升高,LiMn204颗粒的尺寸增大.在焙烧前进行300℃的预处理可显着提高产物中LiMn204相的纯度,同时减小颗粒尺寸和品格常数.3)在300℃预处理,750℃焙烧时,LiMn204颗粒的平均尺寸约为30nIn,锰的平均化合价为3.498与理论值吻合.参考文献:[1]Y un-SungLee,Naokil(1lmada,MasakiY oshio.PowerSoo/~,2∞l, 96:376—384.[2jSeung-TaekMyung,Hoon-B’cIⅡng.PowerSt),l999,84:32—38. [3jHisayukiY amane,TakaoInoue,MihoFujita,et.Poweres,2∞l.99:60.[4]KiSOoPark,MyungHunCho,SangHoPark,eto1.Eloeux~himicaAc- ta.20lD2.47:2937—2942.[5]WangGX,BradhurstDH,LiuHK,et.SolidStateIonics,1999,l20:95—101.[6]Kwang-TaekHwang,Woo-SikUm,Hoe-s(‘0Lee,eto1.PowerSources, l998.74:l69—174.[7]Chung-HsinLu,Shang-wei.PowerSotm:es,2001,97—98:458.18jBarbouxP,TarasconJM,Sh&odliFK.SolidState0m,l99l,94:185.[9]ChoyJ.一H,KimD.-H,Kwonc.-W,et.PowerSoo/~,1999,77:l一11.。
二叔丁基过氧化物msds

企业标识: 企业名称: 企业地址: 邮政编码: 电话号码: 传真号码: 电子邮件:
江苏强盛功能化学股份有限公司 中国江苏常熟市白茆工业经济开发区 215532 +86-512-52533868 +86-512-52537768 mailadmin@
应急咨询电话: +86-532-83889090(24h)
作业人员防护措施、防护装备和应急处置程序: 避免吸入烟雾/蒸汽。避免接触皮肤和眼睛。使用个人防护设备(参见第 8 部分)。
环境保护措施: 不得排放进入排水沟及水源。
泄漏化学品的收容、清除方法及所使用的处置材料: 如果可以,及时切断泄漏源。隔离任何明火,防止火焰、火花产生。采取防静电措施。 用惰性的吸收剂(例如蛭石)覆盖泄露物,可先使其湿润以免扬尘。然后将泄漏物收集 于清洁的容器中再使用(适宜的情况下)或处置。废弃物需受到限制。用大量清水及肥 皂液冲洗污染处。
修订日期 2016-1-11
吸 入: 皮肤接触: 眼睛接触: 食 入:
万一发生吸入性事故,将患者移至新鲜空气处并保持安静。如果呼吸困难, 进行人工呼吸。若可行的话,由医务人员供给氧气。如果症状持续存在, 应立即就医。 立即用大量水冲洗皮肤,脱去受污染的衣物,彻底清洗后才可使用。彻底 清洗或销毁受污染的衣物。如果症状持续存在,应立即进行医治。 首先用大量水冲洗几分钟,如果方便取下隐形眼镜,然后应立即进行医治。 在冲洗的过程中分开眼睑,保证冲洗到眼睛表面及眼睑。如果症状持续存 在,应立即进行医治。 及时呼叫毒物控制中心或医生。若患者是有意识和清醒的,按医生指导立 即引吐。 切勿向失去意识的伤者口中塞任何东西。
5 / 12
化学品安全技术说明书
根据 GB/T 16483-2008、GB/T 17519-2013 编制
过氧化-2-乙基己基单碳酸叔丁酯
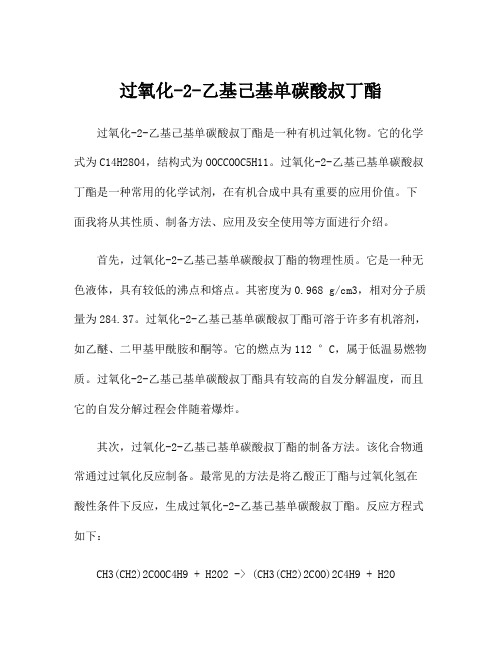
过氧化-2-乙基己基单碳酸叔丁酯过氧化-2-乙基己基单碳酸叔丁酯是一种有机过氧化物。
它的化学式为C14H28O4,结构式为OOCCOOC5H11。
过氧化-2-乙基己基单碳酸叔丁酯是一种常用的化学试剂,在有机合成中具有重要的应用价值。
下面我将从其性质、制备方法、应用及安全使用等方面进行介绍。
首先,过氧化-2-乙基己基单碳酸叔丁酯的物理性质。
它是一种无色液体,具有较低的沸点和熔点。
其密度为0.968 g/cm3,相对分子质量为284.37。
过氧化-2-乙基己基单碳酸叔丁酯可溶于许多有机溶剂,如乙醚、二甲基甲酰胺和酮等。
它的燃点为112 °C,属于低温易燃物质。
过氧化-2-乙基己基单碳酸叔丁酯具有较高的自发分解温度,而且它的自发分解过程会伴随着爆炸。
其次,过氧化-2-乙基己基单碳酸叔丁酯的制备方法。
该化合物通常通过过氧化反应制备。
最常见的方法是将乙酸正丁酯与过氧化氢在酸性条件下反应,生成过氧化-2-乙基己基单碳酸叔丁酯。
反应方程式如下:CH3(CH2)2COOC4H9 + H2O2 -> (CH3(CH2)2COO)2C4H9 + H2O这个反应需要在较低的温度和酸性条件下进行,一般在0-5 °C的范围内。
同时,反应过程需要进行搅拌以提高反应效果。
值得注意的是,过氧化-2-乙基己基单碳酸叔丁酯的制备过程中需要注意安全,避免与热源和明火接触。
过氧化-2-乙基己基单碳酸叔丁酯在有机合成中具有广泛的应用。
它是一种常用的过氧化剂,可以用于氧化反应、氧化脱硫和氧化环化等反应。
例如,过氧化-2-乙基己基单碳酸叔丁酯可以将烯烃氧化成二醇,并且在大部分情况下可以选择性地氧化顺式烯烃。
此外,它还可以用于合成酮、酸和醚等有机化合物。
过氧化-2-乙基己基单碳酸叔丁酯的应用领域还包括颜料、涂料、塑料和聚合物等领域。
最后,我们来谈一下过氧化-2-乙基己基单碳酸叔丁酯的安全使用。
由于它是一种易燃物质且具有爆炸性,所以在使用过程中需要格外注意安全。
二硫化四乙基秋兰姆液相方法

二硫化四乙基秋兰姆液相方法
二硫化四乙基秋兰姆,也被称为促进剂TETD,是一种有机化合物,化学式为C10H20N2S4。
这种化合物具有白色或淡黄色结晶性固体,熔点为70-74℃,沸点为117℃,相对密度为1.27。
二硫化四乙基秋兰姆是一种橡胶硫化促进剂,属于秋兰姆类橡胶硫化促进剂。
这种促进剂焦烧快,硫化速度也快,属于超速硫化促进剂。
硫化平坦性虽小,但能得到抗张强度、定伸强度和硬度高的硫化橡胶。
因此,它可用作二烯类橡胶的助硫化促进剂或无硫磺硫化剂,也可作为低不饱和橡胶的硫化促进剂。
此外,二硫化四乙基秋兰姆还有其他用途。
例如,它可以作为天然橡胶、丁苯橡胶、丁腈橡胶、丁基橡胶、顺丁橡胶及胶乳的超促进剂和硫化剂,还可作为杀菌剂和杀虫剂使用。
在医疗领域,它也常被用作抗酒精中毒的药物。
然而,二硫化四乙基秋兰姆也具有一定的危险性。
根据世界卫生组织国际癌症研究机构的致癌物清单初步整理参考,该物质被归类为3类致癌物。
此外,应避免直接接触这种化合物,以防止可能对皮肤造成的刺激和伤害。
二硫化四乙基秋兰姆是一种重要的化学物质,在多个领域都有广泛的应用。
然而,由于其潜在的危险性,应谨慎处理和使用这种物质。
如需更多关于二硫化四乙基秋兰姆液相
方法的详细信息,建议咨询化学领域专业人士或查阅相关文献资料。
邻苯二甲酸二辛脂MSDS

邻苯二甲酸二辛脂 DOP MSDS第一部分:化学品名称化学品中文名称:邻苯二甲酸二辛酯化学品英文名称:dioctyl phthalate中文名称2:邻苯二甲酸二正辛酯英文名称2:n-octyl phthalate技术说明书编码:2509CAS No.:117-84-0分子式:C24H38O4分子量:390.62第二部分:成分/组成信息有害物成分含量CAS No.邻苯二甲酸二辛酯117-84-0第三部分:危险性概述危险性类别:侵入途径:健康危害:摄入有毒。
对眼睛和皮肤有刺激作用。
受热分解释出腐蚀性、刺激性的烟雾。
环境危害:对环境有危害。
燃爆危险:本品可燃,具刺激性。
第四部分:急救措施皮肤接触:脱去污染的衣着,用大量流动清水冲洗。
眼睛接触:提起眼睑,用流动清水或生理盐水冲洗。
就医。
吸入:迅速脱离现场至空气新鲜处。
保持呼吸道通畅。
如呼吸困难,给输氧。
如呼吸停止,立即进行人工呼吸。
就医。
食入:饮足量温水,催吐。
就医。
第五部分:消防措施危险特性:遇明火、高热可燃。
与氧化剂可发生反应。
流速过快,容易产生和积聚静电。
若遇高热,容器内压增大,有开裂和爆炸的危险。
有害燃烧产物:一氧化碳、二氧化碳。
灭火方法:消防人员须佩戴防毒面具、穿全身消防服,在上风向灭火。
尽可能将容器从火场移至空旷处。
喷水保持火场容器冷却,直至灭火结束。
处在火场中的容器若已变色或从安全泄压装置中产生声音,必须马上撤离。
灭火剂:雾状水、泡沫、干粉、二氧化碳、砂土,不宜用水。
第六部分:泄漏应急处理应急处理:迅速撤离泄漏污染区人员至安全区,并进行隔离,严格限制出入。
切断火源。
建议应急处理人员戴自给式呼吸器,穿一般作业工作服。
不要直接接触泄漏物。
尽可能切断泄漏源。
防止流入下水道、排洪沟等限制性空间。
小量泄漏:用砂土、蛭石或其它惰性材料吸收。
大量泄漏:构筑围堤或挖坑收容。
用泵转移至槽车或专用收集器内,回收或运至废物处理场所处置。
第七部分:操作处置与储存操作注意事项:密闭操作,局部排风。
2,4-二氯过氧化苯甲酰安全技术说明书(msds)
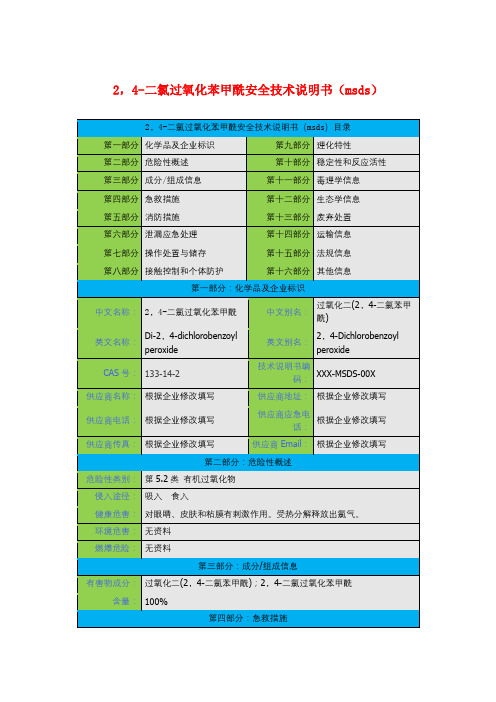
爆炸下限%(V/V):
无资料
外观与性状:
白色至浅黄色结晶粉末或片状带滑感的粉末。
主要用途:
用作硅橡胶硫化剂。
其它理化性质:
无资料
第十部分:稳定性和反应活性
稳定性:
稳定
禁配物:
氧化剂、易燃或可燃物。
避免接触的条件:
无资料
聚合危害:
不能出现
分解产物:
无资料
第十一部分:毒理学信息
急性毒性:
LD50:225mg/kg(小鼠腹腔)LC50:
眼睛防护:
戴安全防护眼镜。
身体防护:
穿防静电工作服。
手防护:
戴防化学品手套。
其他防护:
无资料
第九部分:理化特性
pH:
无资料
熔点(℃):
无资料
沸点(℃):
无资料
分子式:
C14H6Cl4O4
主要成分:
无资料
临界温度(℃):
分解温度/℃:110(半衰期1分钟)
辛醇/水分配系数的对数值:
无资料
饱和蒸气压(kPa):
吸入:
脱离现场至空气新鲜处。呼吸困难时给输氧。呼吸停止时,立即进行人工呼吸。就医。
食入:
误服者,口服牛奶、豆浆或蛋,就医。
第五部分:消防措施
危险特性:
强氧化剂。干燥状态下,受摩擦、震动、撞击可引起爆炸。受热剧烈分解发生爆炸。与还原剂、促进剂、有机物、可燃物等接触发生剧烈反应,有燃烧爆炸危险。
建规火险分级:
运输信息
第七部分
操作处置与储存
第十五部分
法规信息
第八部分
接触控制和个体防护
第十六部分
其他信息
第一部分:化学品及企业标识
分等级大微孔、介孔大孔
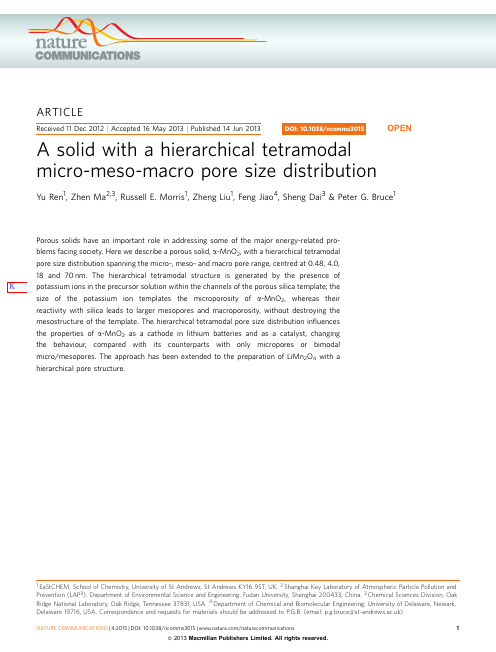
ARTICLEOPENReceived11Dec2012|Accepted16May2013|Published14Jun2013A solid with a hierarchical tetramodalmicro-meso-macro pore size distributionYu Ren1,Zhen Ma2,3,Russell E.Morris1,Zheng Liu1,Feng Jiao4,Sheng Dai3&Peter G.Bruce1Porous solids have an important role in addressing some of the major energy-related pro-blems facing society.Here we describe a porous solid,a-MnO2,with a hierarchical tetramodalpore size distribution spanning the micro-,meso-and macro pore range,centred at0.48,4.0,18and70nm.The hierarchical tetramodal structure is generated by the presence ofpotassium ions in the precursor solution within the channels of the porous silica template;thesize of the potassium ion templates the microporosity of a-MnO2,whereas theirreactivity with silica leads to larger mesopores and macroporosity,without destroying themesostructure of the template.The hierarchical tetramodal pore size distribution influencesthe properties of a-MnO2as a cathode in lithium batteries and as a catalyst,changingthe behaviour,compared with its counterparts with only micropores or bimodalmicro/mesopores.The approach has been extended to the preparation of LiMn2O4with ahierarchical pore structure.1EaStCHEM,School of Chemistry,University of St Andrews,St Andrews KY169ST,UK.2Shanghai Key Laboratory of Atmospheric Particle Pollution and Prevention(LAP3),Department of Environmental Science and Engineering,Fudan University,Shanghai200433,China.3Chemical Sciences Division,Oak Ridge National Laboratory,Oak Ridge,T ennessee37831,USA.4Department of Chemical and Biomolecular Engineering,University of Delaware,Newark,Delaware19716,USA.Correspondence and requests for materials should be addressed to P.G.B.(email:p.g.bruce@).P orous solids have an important role in addressing some of the major problems facing society in the twenty-first century,such as energy storage,CO2sequestration,H2 storage,therapeutics(for example,drug delivery)and catalysis1–8. The size of the pores and their distribution directly affect their ability to function in a particular application2.For example, zeolites are used as acid catalysts in industry,but their micropores impose severe diffusion limitations on the ingress and egress of the reactants and the catalysed products9.To address such issues, great effort is being expended in preparing porous materials with a bimodal(micro and meso)pore structure by synthesizing zeolites or silicas containing micropores and mesopores10–17,or microporous metal–organic frameworks with ordered mesopores18.Among porous solids,porous transition metal oxides are particularly important,because they exhibit many unique properties due to their d-electrons and the variable redox state of their internal surfaces8,19–22.Here we describe thefirst solid(a-MnO2)possessing hierarchical pores spanning the micro,meso and macro range, centred at0.48,4.0,18and70nm.The synthesis method uses mesoporous silica as a hard template.Normally such a template generates a mesoporous solid with a unimodal23–31or,at most,a bimodal pore size distribution32–38.By incorporating Kþions in the precursor solution,within the silica template,the Kþions act bifunctionally:their size templates the formation of the micropores in a-MnO2,whereas their reactivity with silica destroys the microporous channels in KIT-6comprehensively, leading to the formation of a-MnO2containing large mesopores and,importantly,macropores,something that has not been possible by other methods.Significantly,this is achieved without destroying the silica template by alkaline ions.The effect of the tetramodal pore structure on the properties of the material is exemplified by considering their use as electrodes for lithium-ion batteries and as a catalyst for CO oxidation and N2O decomposition.The novel material offers new possibilities for combining the selectivity of small pores with the transport advantages of the large pores across a wide range of sizes.We also present results demonstrating the extension of the method to the synthesis of LiMn2O4with a hierarchical pore structure.ResultsComposition of tetramodal a-MnO2.The composition of the synthesized material was determined by atomic absorption ana-lysis and redox titration to be K0.08MnO2(the K/Mn ratio of the precursor solution was1/3).The material is commonly referred to as a-MnO2,because of the small content of Kþ19.N2sorption analysis of tetramodal a-MnO2.The tetramodal a-MnO2shows a type IV isotherm(Fig.1a).The pore size dis-tribution(Fig.1b)in the range of0.3–200nm was analysed using the density functional theory(DFT)method applied to the adsorption branch of the isotherm39–42,as this is more reliable than analysing the desorption branch43;note that this is not the DFT method used in ab initio electronic structure calculations. Plots were constructed with vertical axes representing ‘incremental pore volume’and‘incremental surface area’.Large (macro)pores can account for a significant pore volume while representing a relatively smaller surface area and vice versa for small(micro)pores.Therefore,when investigating a porous material with a wide range of pore sizes,for example,micropore and macropore,the combination of surface area and pore volume is essential to determine the pore size distribution satisfactorily (Fig.1b).Considering both pore volume and surface area, significant proportions of micro-,meso-and macropores are evident,with distinct maxima centred at0.70,4.0,18and70nm.To probe the size of the micropores more precisely than is possible with DFT,the Horvath–Kawazoe pore size distribution analysis was employed44.A single peak was obtained at0.48nm(Fig.1c),in good accord with the0.46-nm size of the2Â2channels of a-MnO2 (refs.19,21).The relatively small Brunauer–Emmett–Teller(BET) surface area of tetramodal a-MnO2(79–105m2gÀ1; Supplementary Table S1)compared with typical surface areas of mesoporous metal oxides(90–150m2gÀ1)45is due to the significant proportion of macropores(which have small surface areas)and relatively large(18nm)mesopores—a typical mesoporous metal oxide has only3–4nm pores.TEM analysis of tetramodal a-MnO2.Transmission electron microscopic(TEM)data for tetramodal a-MnO2,Fig.2, demonstrates a three-dimensional pore structure with a sym-metry consistent with space group Ia3d.From the TEM data,an a0lattice parameter of23.0nm for the mesostructure could be extracted,which is in good agreement with the value obtained from the low-angle powder X-ray diffraction(PXRD)data, a0¼23.4nm(Supplementary Fig.S1a).High-resolution TEM images in Fig.2c–e demonstrate that the walls are crystalline with a typical wall thickness of10nm.The lattice spacings of0.69,0.31 and0.35nm agree well with the values of6.92,3.09and3.46Åfor the[110],[310]and[220]planes of a-MnO2(International Centre for Diffraction Data(ICDD)number00-044-0141), respectively.The wide-angle PXRD data matches well with the PXRD data of bulk cryptomelane a-MnO2(Supplementary Fig. S1b),confirming the crystalline walls.The various pores in tetramodal a-MnO2can be observed by TEM directly:the0.48-nm micropores are seen in Fig.2e(2Â2 tunnels with dimensions of0.48Â0.48nm in the white box);the 4.0-nm pores are shown in Fig.2b–d;the18-nm pores are shown in Fig.2a;the70-nm pores are evident in Fig.2b(highlighted with white circles).Li intercalation.Li can be intercalated into bulk a-MnO2 (ref.46).Therefore,it is interesting to compare Li intercalation into bulk a-MnO2(micropores only)and bimodal a-MnO2 (micropores along with a single mesopore of diameter3.6nm,see Methods)with tetramodal a-MnO2(micro-,meso-and macropores).Each of the three a-MnO2materials was subjected to Li intercalation by incorporation as the positive electrode in a lithium battery,along with a lithium anode and a non-aqueous electrolyte(see Methods).The results of cycling(repeated intercalation/deintercalation of Li)the cells are shown in Fig.3. Although all exhibit good capacity to cycle Li at low rates of charge/discharge(30mA gÀ1),tetramodal a-MnO2shows sig-nificantly higher capacity(Li storage)at a high rate of 6,000mA gÀ1(corresponding to charge and discharge in3min). The tetramodal a-MnO2can store three times the capacity(Li) compared with bimodal a-MnO2,and18times that of a-MnO2 with only micropores,at the high rate of intercalation/deinter-calation(Fig.3).The superior rate capability of tetramodal a-MnO2over microporous and bimodal forms may be assigned to better Liþtransport in the electrolyte within the hierarchical pore structure of tetramodal a-MnO2.The importance of elec-trolyte transport in porous electrodes has been discussed recently35,47,48and the results presented here reinforce the beneficial effect of a hierarchical pore structure.Catalytic studies.CO oxidation and N2O decomposition were used as reactions to probe the three different forms of a-MnO2as catalysts(Supplementary Fig.S2).As shown in Supplementary Fig.S2a,tetramodal a-MnO2demonstrates better catalytic activity compared with only micropores or bimodal a-MnO2;thetemperature of half CO conversion (T 50)was 124°C for tetra-modal a -MnO 2,whereas microporous and bimodal a -MnO 2exhibited a T 50value of 275°C and 209°C,respectively.In the case of N 2O decomposition,a -MnO 2with only micropores demonstrated no catalytic activity in the range of 200–400°C,in accord with a previous report 49.Tetramodal and bimodal a -MnO 2showed catalytic activity and reached 32%and 20%of N 2O conversion,respectively,at a reaction temperature of 400°C.The differences in catalytic activity are related to the differences in the material.A detailed study focusing on the catalytic activity alonewould be necessary to demonstrate which specific features of the textural differences (pore size distribution,average manganese oxidation state,K þand so on)between the different MnO 2materials are responsible for the differences in behaviour.However,the preliminary results shown here do illustrate that such differences exist.Porous LiMn 2O 4.To demonstrate the wider applicability of the synthesis method,LiMn 2O 4with a hierarchical pore structurewas1801601401201008060402000.00.20.40.60.81.0V (c m 3 g –1)Pore diameter (nm)0.0120.0100.0080.0060.0040.0020.000I n c r e m e n t a l p o r e v o l u m e (c m 3 g –1)Pore width (nm)I n c r e m e n t a l s u r f a c e a r e a (m 2 g –1)I n c r e m e n t a l s u r f a c e a r e a (m 2 g –1)P /P 0Figure 1|N 2sorption analysis of tetramodal a -MnO 2.(a )N 2adsorption–desorption isotherms,(b )DFT pore size distribution and (c )Horvath–Kawazoe pore size distribution from N 2adsorption isotherm for tetramodal a -MnO 2.Figure 2|TEM images of tetramodal a -MnO 2.TEM images along (a )[100]direction,showing 18nm mesopores (scale bar,50nm);(b )4.0and 70nm pores (70nm pores are highlighted by white circles;scale bar,100nm);(c –e )high-resolution (HRTEM)images of tetramodal a -MnO 2showing 4.0and 0.48nm pores (scale bar,10nm).Inset is representation of a -MnO 2structure along the c axis,demonstrating the 2Â2micropores as shown in the HRTEM (white box)in e .Purple,octahedral MnO 6;red,oxygen;violet,potassium.synthesized in a way similar to that of tetramodal a -MnO 2.The main difference is the use of LiNO 3instead of KNO 3(see Methods).In this case,Li þreacts with the silica template col-lapsing/blocking the microporous channels in the KIT-6and resulting in the large mesopores and macropores (17and 50nm)in the LiMn 2O 4obtained.The use of Li þinstead of the larger K þdeters the formation of micropores because Li þis too small.TEM analysis illustrates the hierarchical pore structure of LiMn 2O 4(Supplementary Fig.S3):4.0nm pores are evident in Supplementary Fig.S3b;17nm pores in Supplementary Fig.S3a;and 50nm pores in Supplementary Fig.S3b (highlighted with white circles).The d-spacing of 0.47nm in the high-resolution TEM image (Supplementary Fig.S3c)is in good accordance with the values of 0.4655nm for the [111]planes of LiMn 2O 4(ICDD number 00-038-0789)and with the wide-angle PXRD data (Supplementary Fig.S4).The original DFT pore size distribution analysis from N 2sorption (adsorption branch)gives three pore sizes in the range of 1–100nm centred at 4.0,17and 50nm (Supplementary Fig.S5).A more in-depth presentation of the results for LiMn 2O 4will be given in a future paper;preliminary results presented here illustrate that the basic method can be applied beyond a -MnO 2.DiscussionTurning to the synthesis of the tetramodal a -MnO 2,the details are given in the Methods section.Hard templating using silica templates,such as KIT-6,normally gives rise to materials with unimodal or,at most,bimodal mesopore structures,and in the latter case the smaller mesopores dominate over the larger mesopores 8,32,35.Alkali ions are excellent templates for micropores in transition metal oxides 19,21,but they have been avoided in nanocasting from silica templates because of concerns that they would react with and,hence,destroy thesilica20018016014012010080604020D i s c h a r g e c a p a c i t y (m A h g –1)0Cycle numberx in Li x MnO 2Figure 3|Electrochemical behaviour of different a -MnO 2.Capacity retention for tetramodal a -MnO 2cycled at 30(empty blue circles)and 6,000mA g À1(filled blue circles);bulk a -MnO 2cycled at 30(empty red squares)and 6,000mA g À1(filled red squares);bimodal a -MnO 2cycled at 30(empty black triangle)and 6,000mA g À1(filled blacktriangles).18 nm pores70 nm poresTwo sets of mesoporeschannels connecting both sets of mesoporesEtching of silica Etching of silica Etching of silica template2discontinuously within one set of the KIT-6mesoporesFigure 4|Formation mechanism of meso and macropores in tetramodal a -MnO 2.When both KIT-6mesochannels are occupied by a -MnO 2and then the silica between them etched away,the remaining pore is 4nm (centre portion of figure).When a -MnO 2grows in only one set of mesochannels and then the KIT-6is dissolved away,the remaining metal oxide has 18nm pores (upper portion of figure).The comprehensive destruction of the microchannels in KIT-6by K þleads to a -MnO 2growing in only a proportion of one set of the KIT-6mesochannels,resulting in the formation of B 70nm pores (lower portion of figure).template50.Here,not only have alkali ions been used successfully in precursor solutions without destroying the template mesostructure but they give rise to macropores in the a-MnO2, thus permitting the synthesis of a tetramodal,micro-small,meso-large,meso-macro pore structure.Synthesis begins by impregnating the KIT-6silica template with a precursor solution containing Mn2þand Kþions.On heating,the Kþions template the formation of the micropores in a-MnO2,as the latter forms within the KIT-6template.KIT-6 consists of two interpenetrating mesoporous channels linked by microporous channels51–53.The branches of the two different sets of mesoporous channels in KIT-6are nearest neighbours separated by a silica wall of B4nm53;therefore,when both KIT-6mesochannels are occupied by a-MnO2and the silica between them etched away,the remaining pore is4nm(see centre portion of Fig.4).It has been shown previously,by a number of authors,that by varying the hydrothermal conditions used to prepare the KIT-6,the proportion of the microchannels can be decreased to some extent,thus making it difficult to simultaneouslyfill the neighbouring KIT-6mesoporous channels by the precursor solution of the target mesoporous metal oxide33–35.As a result,the target metal oxide grows in only one set of mesochannels of the KIT-6host but not both.When the KIT-6is dissolved away,the remaining metal oxide has B18nm pores,because the distance between adjacent branches of the same KIT-6mesochannels is greater than between the two different mesochannels in KIT-6.Here we propose that the Kþions have a similar effect on the KIT-6to that of the hydrothermal synthesis,but by a completely different mechanism.Reaction between the Kþions in the precursor solution with the silica during calcination results in the formation of Kþ-silicates,which cause collapse or blocking of the microporous channels in KIT-6,such that the a-MnO2grows in one set of the KIT-6mesochannels,giving rise to18nm pores in a-MnO2when the silica is etched away,see top portion of Fig.4. However,the reaction between Kþand the silica is more severe than the effect of varying the hydrothermal treatment.In the former case,the KIT-6microchannels are so comprehensively destroyed that the proportion of the large(18nm)to smaller (4nm)mesopores is greater than can be achieved by varying hydrothermal conditions.The comprehensive destruction of the microchannels in KIT-6by Kþ,perhaps augmented by some minor degradation of parts of the mesochannels,leads to a-MnO2 growing in only a proportion of one set of the KIT-6 mesochannels,resulting in the formation of B70nm pores in a-MnO2,see lower portion of Fig.4.In summary,the Kþreactivity with the silica goes beyond what can be achieved by varying the conditions of hydrothermal synthesis and is responsible for generating the tetramodal pore size distribution reported here. The mechanism of pore formation in a-MnO2by reaction between Kþand the silica template is supported by several findings.First,by the lower K/Mn molar ratio of thefinal tetramodal a-MnO2product(0.08)compared with the starting materials(0.33)implies that some of the Kþions in the impregnating solution have reacted with the silica.Second, support for collapse/blocking of the microporous channels in KIT-6due to reaction with Kþwas obtained by comparing the texture of KIT-6impregnated with an aqueous solution contain-ing only KNO3and calcined at300and500°C.The micropore volume in KIT-6is the greatest,with no KNO3in the solution;it then decreases continuously as the calcination temperature and calcination time is increased,such that after2and5h at500°C the micropore volume has decreased to zero(Supplementary Fig. S6).Third,we prepared tetramodal a-MnO2using a similar synthetic procedure to that described in the Methods section, except that this time we used a covered tall crucible for the calcination step.Sun et al.54have shown that using a covered,tall crucible when calcining results in porous metal oxides with much larger particle sizes.If the70-nm pores had arisen simply from the gaps between the particles,then the pore size would have changed;in contrast,it remained centred at70nm, Supplementary Fig.S7,consistent with the70-nm pores being intrinsic to the materials and arising from reaction with the Kþas described above.Fourth,if the synthesis of MnO2is carried out using the KIT-6template but in the absence Kþions,then the DFT pore size distribution shown in Supplementary Fig.S8is obtained.The0.48-and70-nm pores are now absent,but the4-and18-nm pores remain.This demonstrates the key role of Kþin the formation of the smallest and largest pores and,hence,in generating the tetramodal pore size distribution.The absence of Kþmeans that there is nothing to template the0.48nm pores and so a-MnO2is not formed;the b-polymorph is obtained instead.The absence of Kþalso means that the microchannels in the KIT-6template remain intact,resulting in no70nm pores and the dominance of the4-nm pores compared with the 18-nm pores.The hierarchical pore structure can be varied systematically by controlling the synthesis conditions,in particular the Kþ/Mn ratio of the precursor solution.A range of Kþ/Mn ratios,1/5,1/3and1/2,gave rise to a series of pore size distributions,in which the pore sizes remained the same but the relative proportions of the different pores varied (Supplementary Table S1).The higher the Kþ/Mn ratio,the greater the proportion of macropores and large mesopores.This is in accord with expectations,as the higher the Kþconcentra-tion in the precursor solution the greater the collapse/blocking of the microporous channels in the KIT-6(as noted above),and hence the greater the proportion of macropores and large mesopores.Indeed,these results offer further support for the mechanism of pore size distribution arising from reaction between Kþand the silica template.In conclusion,tetramodal a-MnO2,thefirst porous solid with a tetramodal pore size distribution,has been synthesized.Its hierarchical pore structure spans the micro,meso and macropore range between0.3and200nm,with pore dimensions centred at 0.48,4.0,18and70nm.Key to the synthesis is the use of Kþions that not only template the formation of micropores but also react with the silica template,therefore,breaking/blocking the micro-porous channels in the silica template far more comprehensively than is possible by varying the hydrothermal synthesis conditions, to the extent that macropores are formed,and without destroying the silica mesostructure by alkali ions,as might have been expected.The resulting hierarchical tetramodal structure demon-strates different behaviours compared with microporous and bimodal a-MnO2as a cathode material for Li-ion batteries,and when used as a catalyst for CO oxidation and N2O decomposi-tion.The method has been extended successfully to the preparation of hierarchical LiMn2O4.MethodsSynthesis.Tetramodal a-MnO2(surface area96m2gÀ1,K0.08MnO2)was pre-pared by two-solvent impregnation55using Kþand mesoporous silica KIT-6as the hard template.KIT-6was prepared according to a previous report (hydrothermal treatment at100°C)51.In a typical synthesis of tetramodal a-MnO2, 7.53g of Mn(NO3)2Á4H2O(98%,Aldrich)and1.01g of KNO3(99%,Aldrich)were dissolved in B10ml of water to form a solution with a molar ratio of Mn/K¼3.0. Next,5g of KIT-6was dispersed in200ml of n-hexane.After stirring at room temperature for3h,5ml of the Mn/K solution was added slowly with stirring.The mixture was stirred overnight,filtered and dried at room temperature until a completely dried powder was obtained.The sample was heated slowly to500°C (1°C minÀ1),calcined at that temperature for5h with a cover in a normal crucible unless is specified54and the resulting material treated three times with a hot aqueous KOH solution(2.0M),to remove the silica template,followed by washing with water and ethanol several times,and then drying at60°C.Bimodal a-MnO2(surface area58m2gÀ1,K0.06MnO2)with micropore and a single mesopore size of3.6nm was prepared by using mesoporous silica SBA-15as a hard template.The SBA-15was prepared according to a previous report56.Bulk a-MnO2(surface area8m2gÀ1,K0MnO2)was prepared by the reaction between325mesh Mn2O3(99.0%,Aldrich)and6.0M H2SO4solution at80°C for 24h,resulting in the disproportionation of Mn2O3into a soluble Mn2þspecies and the desired a-MnO2product46.Treatment of KIT-6with KNO3was carried out as follows:1.01g of KNO3was dissolved in B15ml of water to form a KNO3solution.Five grams of mesoporous KIT-6was dispersed in200ml of n-hexane.After stirring at room temperature for 3h,5ml of KNO3solution was added slowly with stirring.The mixture was stirred overnight,filtered and dried at room temperature until a completely dried powder was obtained.The sample was heated slowly to300or500°C(1°C minÀ1), calcined at that temperature for5h and the resulting material was washed with water and ethanol several times,and then dried at60°C overnight.The synthesis method for hierarchical porous LiMn2O4was similar to that of tetramodal a-MnO2.The main difference was to use1.01g of LiNO3instead of KNO3.After impregnation into KIT-6,calcination and silica etching,porous LiMn2O4was obtained.Characterization.TEM studies were carried out using a JEOL JEM-2011, employing a LaB6filament as the electron source,and an accelerating voltage of 200keV.TEM images were recorded by a Gatan charge-coupled device camera in a digital format.Wide-angle PXRD data were collected on a Stoe STADI/P powder diffractometer operating in transmission mode with Fe K a1source radiation(l¼1.936Å).Low-angle PXRD data were collected using a Rigaku/MSC,D/max-rB with Cu K a1radiation(l¼1.541Å)operating in reflection mode with a scintillation detector.N2adsorption–desorption analysis was carried out using a Micromeritics ASAP2020.The typical sample weight used was100–200mg. The outgas condition was set to300°C under vacuum for2h,and all adsorption–desorption measurements were carried out at liquid nitrogen tem-perature(À196°C).The original DFT method for the slit pore geometry was used to extract the pore size distribution from the adsorption branch usingthe Micromeritics software39–42.A Horvath–Kawazoe method was used to extract the microporosity44.Mn and K contents were determined by chemical analysis using a Philips PU9400X atomic adsorption spectrometer.The average oxidation state of framework manganese in a-MnO2samples was determined by a redoxtitration method57.Electrochemistry.First,the cathode was constructed by mixing the active material (a-MnO2),Kynar2801(a copolymer based on polyvinylidenefluoride),and Super S carbon(MMM)in the weight ratio80:10:10.The mixture was cast onto Al foil (99.5%,thickness0.050mm,Advent Research Materials,Ltd)from acetone using a Doctor-Blade technique.After solvent evaporation at room temperature and heating at80°C under vacuum for8h,the cathode was assembled into cells along with a Li metal anode and electrolyte(Merck LP30,1M LiPF6in1:1v/v ethylene carbonate/dimethyl carbonate).The cells were constructed and handled in anAr-filled MBraun glovebox(O2o0.1p.p.m.,H2O o0.1p.p.m.).Electrochemical measurements were carried out at30°C using a MACCOR Series4200cycler.Catalysis.Catalytic CO oxidation was tested in a plug-flow microreactor(Alta-mira AMI200).Fifty milligrams of catalyst was loaded into a U-shaped quartz tube (4mm i.d.).After the catalyst was pretreated inflowing8%O2(balanced with He) at400°C for1h,the catalyst was then cooled down,the gas stream switched to1% CO(balanced with air)and the reaction temperature ramped using a furnace(at a rate of1°C minÀ1above ambient temperature)to record the light-off curve.The flow rate of the reactant stream was37cm3minÀ1.A portion of the product stream was extracted periodically with an automatic sampling valve and was analysed using a dual column gas chromatograph with a thermal conductivity detector.To perform N2O decomposition reaction testing,0.5g catalyst was packed into a U-shaped glass tube(7mm i.d.)sealed by quartz wool,and pretreated inflowing 20%O2(balance He)at400°C for1h(flow rate:50cm3minÀ1).After cooling to near-room temperature,a gas stream of0.5%N2O(balance He)flowed through the catalyst at a rate of60cm3minÀ1,and the existing stream was analysed by a gas chromatograph(Agilent7890A)that separates N2O,O2and N2.The reaction temperature was varied using a furnace,and kept at100,150,200,250,300,350 and400°C for30min at each reaction temperature.The N2O conversion determined from GC analysis was denoted as X¼([N2O]in—[N2O]out)/[N2O]inÂ100%.References1.Corma,A.From microporous to mesoporous molecular sieve materials andtheir use in catalysis.Chem.Rev.97,2373–2419(1997).2.Davis,M.E.Ordered porous materials for emerging applications.Nature417,813–821(2002).3.Taguchi,A.&Schu¨th,F.Ordered mesoporous materials in catalysis.Micro.Meso.Mater.77,1–45(2005).4.Fe´rey,G.Hybrid porous solids:past,present,future.Chem.Soc.Rev.37,191–214(2008).5.Bruce,P.G.,Scrosati,B.&Tarascon,J.M.Nanomaterials for rechargeablelithium batteries.Angew.Chem.Int.Ed.47,2930–2946(2008).6.Zhai,Y.et al.Carbon materials for chemical capacitive energy storage.Adv.Mater.23,4828–4850(2011).7.Tu¨ysu¨z,H.&Schu¨th,F.in Advances in Catalysis.Chapter Two Vol.55pp127–239(Academic Press,2012).8.Ren,Y.,Ma,Z.&Bruce,P.G.Ordered mesoporous metal oxides:synthesis andapplications.Chem.Soc.Rev.41,4909–4927(2012).9.Corma,A.State of the art and future challenges of zeolites as catalysts.J.Catal.216,298–312(2003).10.Liu,Y.,Zhang,W.&Pinnavaia,T.J.Steam-stable aluminosilicatemesostructures assembled from zeolite type Y seeds.J.Am.Chem.Soc.122, 8791–8792(2000).11.Meng,X.J.,Nawaz,F.&Xiao,F.S.Templating route for synthesizingmesoporous zeolites with improved catalytic properties.Nano Today4,292–301(2009).12.Lopez-Orozco,S.,Inayat,A.,Schwab,A.,Selvam,T.&Schwieger,W.Zeoliticmaterials with hierarchical porous structures.Adv.Mater.23,2602–2615(2011).13.Na,K.et al.Directing zeolite structures into hierarchically nanoporousarchitectures.Science333,328–332(2011).14.Chen,L.-H.et al.Hierarchically structured zeolites:synthesis,mass transportproperties and applications.J.Mater.Chem.22,17381–17403(2012).15.Tsapatsis,M.Toward high-throughput zeolite membranes.Science334,767–768(2011).16.Zhang,X.et al.Synthesis of self-pillared zeolite nanosheets by repetitivebranching.Science336,1684–1687(2012).17.Jiang,J.et al.Synthesis and structure determination of the hierarchicalmeso-microporous zeolite ITQ-43.Science333,1131–1134(2011).18.Zhao,Y.et al.Metal–organic framework nanospheres with well-orderedmesopores synthesized in an ionic liquid/CO2/surfactant system.Angew.Chem.Int.Ed.50,636–639(2011).19.Feng,Q.,Kanoh,H.&Ooi,K.Manganese oxide porous crystals.J.Mater.Chem.9,319–333(1999).20.Tiemann,M.Repeated templating.Chem.Mater.20,961–971(2008).21.Suib,S.L.Structure,porosity,and redox in porous manganese oxide octahedrallayer and molecular sieve materials.J.Mater.Chem.18,1623–1631(2008).22.Zheng,H.et al.Nanostructured tungsten oxide–properties,synthesis,andapplications.Adv.Funct.Mater.21,2175–2196(2011).ha,S.C.&Ryoo,R.Synthesis of thermally stable mesoporous cerium oxidewith nanocrystalline frameworks using mesoporous silica templates.Chem.Commun.39,2138–2139(2003).24.Tian,B.Z.et al.General synthesis of ordered crystallized metal oxidenanoarrays replicated by microwave-digested mesoporous silica.Adv.Mater.15,1370–1374(2003).25.Zhu,K.K.,Yue,B.,Zhou,W.Z.&He,H.Y.Preparation of three-dimensionalchromium oxide porous single crystals templated by mun.39,98–99(2003).26.Tian,B.Z.et al.Facile synthesis and characterization of novel mesoporous andmesorelief oxides with gyroidal structures.J.Am.Chem.Soc.126,865–875 (2004).27.Jiao,F.,Shaju,K.M.&Bruce,P.G.Synthesis of nanowire and mesoporouslow-temperature LiCoO2by a post-templating reaction.Angew.Chem.Int.Ed.44,6550–6553(2005).28.Rossinyol,E.et al.Nanostructured metal oxides synthesized by hard templatemethod for gas sensing applications.Sens.Actuator B Chem.109,57–63(2005).29.Shen,W.H.,Dong,X.P.,Zhu,Y.F.,Chen,H.R.&Shi,J.L.MesoporousCeO2and CuO-loaded mesoporous CeO2:Synthesis,characterization,and CO catalytic oxidation property.Micro.Meso.Mater.85,157–162(2005).30.Wang,Y.Q.et al.Weakly ferromagnetic ordered mesoporous Co3O4synthesized by nanocasting from vinyl-functionalized cubic Ia3d mesoporous silica.Adv.Mater.17,53–56(2005).31.Ren,Y.et al.Ordered crystalline mesoporous oxides as catalysts for COoxidation.Catal.Lett.131,146–154(2009).32.Jiao,K.et al.Growth of porous single-crystal Cr2O3in a3-D mesopore system.mun.41,5618–5620(2005).33.Rumplecker,A.,Kleitz,F.,Salabas,E.L.&Schu¨th,F.Hard templating pathwaysfor the synthesis of nanostructured porous Co3O4.Chem.Mater.19,485–496 (2007).34.Jiao,F.et al.Synthesis of ordered mesoporous NiO with crystalline walls anda bimodal pore size distribution.J.Am.Chem.Soc.130,5262–5266(2008).35.Ren,Y.,Armstrong,A.R.,Jiao,F.&Bruce,P.G.Influence of size on therate of mesoporous electrodes for lithium batteries.J.Am.Chem.Soc.132, 996–1004(2010).。
过氧化二-(2,4-二氯苯甲酰)-理化性质及危险特性表
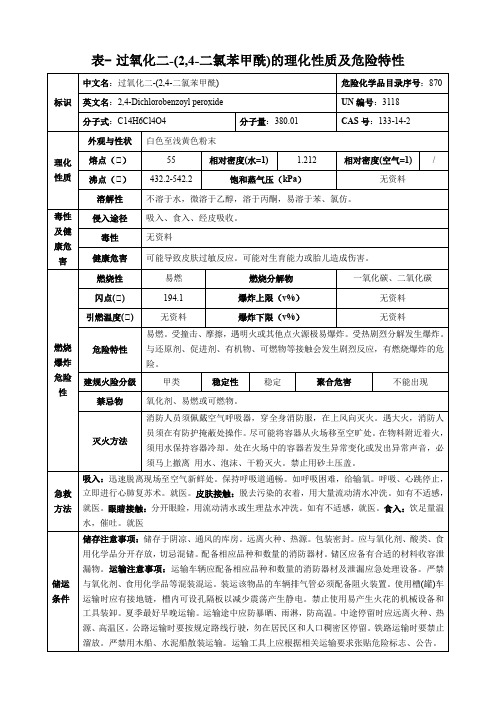
稳定
聚合危害
不能出现
禁忌物
氧化剂、易燃或可燃物。
灭火方法
消防人员须佩戴空气呼吸器,穿全身消防服,在上风向灭火。遇大火,消防人员须在有防护掩蔽处操作。尽可能将容器从火场移至空旷处。在物料附近着火,须用水保持容器冷却。处在火场中的容器若发生异常变化或发出异常声音,必须马上撤离用水、泡沫、干粉灭火。禁止用砂土压盖。
急救方法
吸入:迅速脱离现场至空气新鲜处。保持呼吸道通畅。如呼吸困难,给输氧。呼吸、心跳停止,立即进行心肺复苏术。就医。皮肤接触:脱去污染的衣着,用大量流动清水冲洗。如有不适感,就医。眼睛接触:分开眼睑,用流动清水或生理盐水冲洗。如有不适感,就医。食入:饮足量温水,催吐。就医
储运条件
储存注意事项:储存于阴凉、通风的库房。远离火种、热源。包装密封。应与氧化剂、酸类、食用化学品分开存放,切忌混储。配备相应品种和数量的消防器材。储区应备有合适的材料收容泄漏物。运输注意事项:运输车辆应配备相应品种和数量的消防器材及泄漏应急处理设备。严禁与氧化剂、食用化学品等混装混运。装运该物品的车辆排气管必须配备阻火装置。使用槽(罐)车运输时应有接地链,槽内可设孔隔板以减少震荡产生静电。禁止使用易产生火花的机械设备和工具装卸。夏季最好早晚运输。运输途中应防暴晒、雨淋,防高温。中途停留时应远离火种、热源、高温区。公路运输时要按规定路线行驶,勿在居民区和人口稠密区停留。铁路运输时要禁止溜放。严禁用木船、水泥船散装运输。运输工具上应根据相关运输要求张贴危险标志、公告。
燃烧爆炸危险性
燃烧性
易燃
燃烧分解物
一氧化碳、二氧化碳
闪点(℃)
194.1
爆炸上限(v%)
无资料
引燃温度(℃)
无资料
爆炸下限(v%)
罗氟司特

罗氟 司 特 化 合 物 的 专 利 文 献 有 IN2004MU00478、 WO2005026095、WO2004033430、US6822114。
参考文献:
[1] WILLIAMS E L,WU T. Process for production of fluoroalkoxysubstituted benzamides ( roflumilast and intermediates ) : US, 6822114 B1 [P]. 2004 - 11 - 23.
第 21 卷 第 4 期 2011 年 8 月 总 102 期
中国药物化学杂志 Chinese Journal of M edicinal Chemistry
文章编号: 1005 - 0108( 2011) 04 - 0332 - 01
罗氟司特( roflumilas No. 4 p. 332 Aug. 2011 Sum 102
檨檨檨檨殎 新药信息
檨殎
罗氟司特( roflumilast) 是由 Forest Lab 的子公司 Forest Pharmaceuticals 生产上市的磷酸二酯酶-4 ( PDE-4) 抑 制剂,商品名 Daxas。2011 年 2 月 28 日,roflumilast 经美 国 FDA 批准上市,用于慢性阻塞性肺炎( COPD) 的治疗。 罗氟司特的中文化学名称: 3-( 环丙基甲氧基) -N-( 3,5-二 氯吡啶-4-基) -4-( 二氟甲氧基) 苯甲酰胺; 英文化学名称: N-( 3,5-dichloropyridin-4-yl ) -3-cyclopropylmethoxy-4-difluoromethoxybenzamide; 分 子 式: C17 H14 Cl2F2N2O3; 分 子 量: 403. 22; CAS 登记号: 162401-32-3。
过氧化二-(2,4-二氯苯甲酰)[糊状物,含量≤52%]安全技术说明书MSDS
![过氧化二-(2,4-二氯苯甲酰)[糊状物,含量≤52%]安全技术说明书MSDS](https://img.taocdn.com/s3/m/bc1aca6202d276a201292e09.png)
第一部分化学品及企业标识化学品中文名:过氧化二-(2,4-二氯苯甲酰)[糊状物,含量≤52%]化学品英文名:di-(2,4-dichlorobenzoyl)peroxide;2,4,2',4’-tetrachlorobenzoyl peroxideCAS No.:133-14-2EC No.:205-094-9分子式:C14H6Cl4O4第二部分危险性概述| 紧急情况概述固体。
遇热有火灾危险。
| GHS 危险性类别根据GB 30000-2013化学品分类和标签规范系列标准(参阅第十六部分),该产品分类如下:有机过氧化物,E型。
| 标签要素象形图警示词:警告危险信息:加热可能起火。
预防措施:远离热源、热表面、火花、明火以及其它点火源。
禁止吸烟。
只能在原包装中存放。
保持低温。
容器和接收设备接地和等势联接。
戴防护手套/穿防护服/戴防护眼罩/戴防护面具。
事故响应:不适用。
安全储存:存放在通风良好的地方。
防日晒。
分开存放。
废弃处置:按照地方/区域/国家/国际规章处置内装物/容器。
物理化学危险遇热有火灾危险。
健康危害吸入该物质可能会引起对健康有害的影响或呼吸道不适。
意外食入本品可能对个体健康有害。
通过割伤、擦伤或病变处进入血液,可能产生全身损伤的有害作用。
眼睛直接接触本品可导致暂时不适。
环境危害请参阅 SDS 第十二部分。
第三部分成分/组成信息第四部分急救措施皮肤接触:立即脱去污染的衣物。
用大量肥皂水和清水冲洗皮肤。
如有不适,就医。
眼睛接触:用大量水彻底冲洗至少 15 分钟。
如有不适,就医。
吸入:立即将患者移到新鲜空气处,保持呼吸畅通。
如果呼吸困难,给于吸氧。
如患者食入或吸入本物质,不得进行口对口人工呼吸。
如果呼吸停止。
立即进行心肺复苏术。
立即就医。
食入:禁止催吐,切勿给失去知觉者从嘴里喂食任何东西。
立即呼叫医生或中毒控制中心。
第五部分消防措施| 危险特性加热时,容器可能爆炸。
暴露于火中的容器可能会通过压力安全阀泄漏出内容物。
二乙氧基膦酰乙酸叔丁酯的热裂解反应有机化学

二乙氧基膦酰乙酸叔丁酯的热裂解反应有机化学二乙氧基膦酰乙酸叔丁酯的热裂解是一个典型的消除反应,其反应机理如下:
首先,通过热量输入,二乙氧基膦酰乙酸叔丁酯将经历一个活化过程,激发分子内部的化学键振动能,从而变得更加容易分解。
接下来,在分子内部的羰基部位和邻位的乙氧基之间发生酯水解反应,生成一个乙酸叔丁酯分子和一个膦酸二乙酯酯分子。
然后,由于膦酸二乙酯重组不容易发生,分子内部的另一个乙氧基将与膦酸二乙酯分子中的羰基部位形成氧膦键,这个过程同时伴随着一个乙醇分子的析出,使得产生了乙烯和二甲基膦酸二乙酯两个反应产物。
综合来看,这个反应的总的化学方程式为:
C13H25O5P →C8H16 + C2H5O2C(CH3)3 + (CH3)2PO(OH)2 + C2H5OH
其中分别代表二乙氧基膦酰乙酸叔丁酯、乙烯、乙酸叔丁酯、二甲基膦酸二乙酯和乙醇。
茂金属的制备方法[发明专利]
![茂金属的制备方法[发明专利]](https://img.taocdn.com/s3/m/274d80d267ec102de2bd89fc.png)
专利名称:茂金属的制备方法
专利类型:发明专利
发明人:A·L·沙巴诺维,E·M·拉玛泽诺瓦申请号:CN200680047852.X
申请日:20061227
公开号:CN101341164A
公开日:
20090107
专利内容由知识产权出版社提供
摘要:公开了一种用于生产二聚环戊二烯铁及其他环戊二烯有机金属化合物的方法。
在干燥的氮气条件下,在冷却的烧瓶中,向环戊二烯、二乙胺、一种基团1A金属和冠醚的溶液中加入干燥的氯化亚铁(或者其他选择的金属盐)。
剧烈混合该溶液几小时并蒸发掉任何过量的二乙胺。
使用石油醚从剩余物中提取出二聚环戊二烯铁(或者其他的环戊二烯有机金属化合物),且除去所有溶剂。
申请人:普罗佩蒂发展国际有限公司
地址:巴拿马巴库
国籍:PA
代理机构:永新专利商标代理有限公司
代理人:过晓东
更多信息请下载全文后查看。
脂肪二酸单叔丁酯
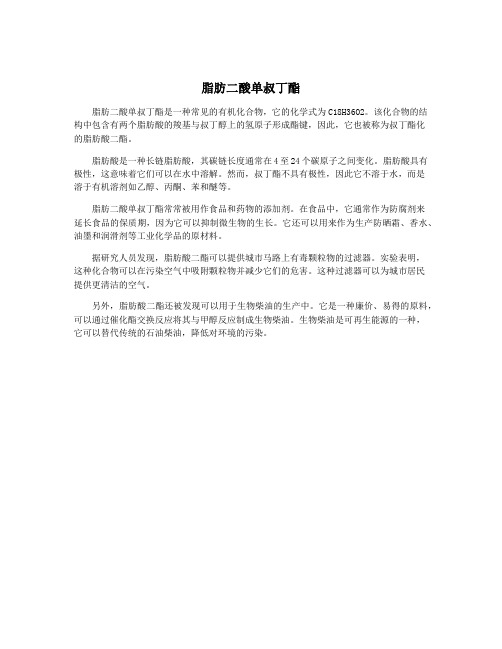
脂肪二酸单叔丁酯
脂肪二酸单叔丁酯是一种常见的有机化合物,它的化学式为C18H36O2。
该化合物的结构中包含有两个脂肪酸的羧基与叔丁醇上的氢原子形成酯键,因此,它也被称为叔丁酯化
的脂肪酸二酯。
脂肪酸是一种长链脂肪酸,其碳链长度通常在4至24个碳原子之间变化。
脂肪酸具有极性,这意味着它们可以在水中溶解。
然而,叔丁酯不具有极性,因此它不溶于水,而是
溶于有机溶剂如乙醇、丙酮、苯和醚等。
脂肪二酸单叔丁酯常常被用作食品和药物的添加剂。
在食品中,它通常作为防腐剂来
延长食品的保质期,因为它可以抑制微生物的生长。
它还可以用来作为生产防晒霜、香水、油墨和润滑剂等工业化学品的原材料。
据研究人员发现,脂肪酸二酯可以提供城市马路上有毒颗粒物的过滤器。
实验表明,
这种化合物可以在污染空气中吸附颗粒物并减少它们的危害。
这种过滤器可以为城市居民
提供更清洁的空气。
另外,脂肪酸二酯还被发现可以用于生物柴油的生产中。
它是一种廉价、易得的原料,可以通过催化酯交换反应将其与甲醇反应制成生物柴油。
生物柴油是可再生能源的一种,
它可以替代传统的石油柴油,降低对环境的污染。
- 1、下载文档前请自行甄别文档内容的完整性,平台不提供额外的编辑、内容补充、找答案等附加服务。
- 2、"仅部分预览"的文档,不可在线预览部分如存在完整性等问题,可反馈申请退款(可完整预览的文档不适用该条件!)。
- 3、如文档侵犯您的权益,请联系客服反馈,我们会尽快为您处理(人工客服工作时间:9:00-18:30)。
LiMn2O4cathode doped with excess lithium and synthesizedby co-precipitation for Li-ion batteriesH.W.Chan a,J.G.Duh a,*,S.R.Sheen ba Department of Materials Science and Engineering,National Tsing Hua University,Hsinchu,Taiwanb Coremax Taiwan Corporation,Hsinchu Hsien,TaiwanReceived20August2002;accepted9November2002AbstractLiMn2O4exhibits lower cost,acceptable environmental characteristics,and better safety properties than other positive-electrode(cathode) materials for lithium-ion batteries.In this study,excess Li doped Li1þx Mn2O4is synthesized by a well-mixed co-precipitation method with LiOH utilized as both the reactant and co-precipitation agent.The precursor is calcined for various heating times and temperatures to form a fine powder of a single spinel phase with different particle sizes,size distributions,and morphology.The minimum heating temperature is around4008C.For short heating periods,Mn2O3impurity is observed,but disappears after longer heating times.The average particle size is in the range2–8m m for powders calcined between700and8708C.The lattice parameter increases with increase in heating temperature.The electrochemical behavior of LiMn2O4powder is examined by using test cells which consist of a cathode,a metallic lithium anode,and an electrolyte of1M LiPF6in a1:1(volume ratio)mixture of ethylene carbonate(EC)and dimethyl carbonate(DMC).Cells with cathodes of LiMn2O4,Li1.08Mn2O4and Li1.1Mn2O4give a capacity of85,109and126mAh gÀ1,respectively.The introduction of excess Li in LiMn2O4 apparently increases the capacity,and decreases significantly the rate of capacity degradation on charge–discharge cycling.#2002Elsevier Science B.V.All rights reserved.Keywords:Lithium-ion battery;Cathode material;Co-precipitation method;Excess lithium;LiMn2O4;Capacity1.IntroductionThe positive-electrode(cathode)material plays a critical role in the performance of Li-ion batteries.Accordingly, various kinds of cathode materials of low material cost,high stability and good electrochemical performance have been investigated.Some of candidates are LiMn2O4with a spinel (Fd3m)structure,LiCoO2and LiNiO2with layer(R3m) structure,and LiNiVO4and LiCoVO4[1]with an inverse spinel(Fd3m)structure.The ternary lithium manganese oxide,LiMn2O4,and its related compounds have been studied extensively as a cathode material with three-dimensional framework for Li-ion rechargeable cells.LiMn2O4wasfirst synthesized is1958[2]by heating a mixture of lithium carbonate and manganese oxide at8508C in air.In1981,Hunter[3] reported that lithium ions could be de-intercalated comple-tely from the spinel LiMn2O4structure to form l-MnO2by a chemical procedure[3].Given this performance,Thackeray et ed LiMn2O4as a cathode material in1983[4].The theoretical specific capacity of LiMn2O4is148 mAh gÀ1,and the practical specific capacity approaches 120mAh gÀ1.For some applications,however,a capacity of about120mAh gÀ1is acceptable,provided the value remains stable under extended charge–discharge cycling. During de-intercalation,lithium ions leave the spinel LiMn2O4structure and this leads to the formation of Mn2O4in which the spinel structure is retained.It is supposed that lithium ions can be intercalated fully from the host structure and that the structure does not deteriorate as do LiNiO2and LiCoO2when lithium ions are extracted. Spinel LiMn2O4is usually synthesized at high tempera-tures by a solid-state reaction[5].At lower temperatures, some Mn2O3may be present as an impurity and only disappears at8008C[6–8].Compared with the solid-state method,the stoichiometry of lithium manganese oxide can be controlled more exactly by the co-precipitation method. In this study,a simple modified approach is adopted to provide LiMn2O4with adequate electrochemical properties for use in Li-ion rechargeable batteries.The co-precipitation method is carried out in de-ionized water using LiOH as reactant and a co-precipitation agent.The selection of LiOH decreases the complexity of the co-precipitation methodandJournal of Power Sources115(2003)110–118*Corresponding author.Fax:þ886-3-571-2686.E-mail address:jgd@.tw(J.G.Duh).0378-7753/02/$–see front matter#2002Elsevier Science B.V.All rights reserved.PII:S0378-7753(02)00616-Xallows a more intimate mixing of lithium and manganese in the starting materials,which results in a homogeneous LiMn2O4product with well-controlled morphology.In addi-tion,excess Liþions act as a‘reservoir’to overcome the well-known loss in capacity during thefirst charge of lithium-ion batteries[9,10].2.Experimental2.1.Powder preparationStoichiometric,excess-Li,spinel Li1þx Mn2O4was synthesized from the reaction of a mixture of lithium hydroxide(LiOH)and manganese acetate(Mn(CH3COO)2).A stoichiometric amount of the latter two compounds a cationic ratio of Li:Mn¼ð1þxÞ:2was dissolved in de-ionized water and mixed well by stirring gently.The sample designation is listed in Table1.The solution was evaporated at1008C for10h to obtain the precursor powder.The precursor was preheated at4008C for1h,and then calcined at elevated temperature at a rate of28C/min in air to examine the reaction process for the formation of single-phase LiMn2O4.2.2.Characterization and analysis Thermogravimetry/diffrential thermal analysis(TG/DTA, SSC5000,Seiko,Japan)was employed to determine the preheating and minimum calcine temperature with the heat-ing rate of108C/min from room temperature to10008C. The compositions of the calcined powder were analyzed with an inductively coupled plasma–atomic emission spec-trometer(ICP–AES,Perkin-Elmer,Optima3000DV,USA), and were further determined with an electron probe micro-analyzer(EPMA,JXA-8800M,JEOL,Japan).Since lithium cannot be detected by EPMA,the contents of manganese and oxygen werefirst evaluated by means of the ZAF technique in EPMA and then the amount of lithium was obtained by a difference and normalization approach.The phase composition and crystal structure of the heat-treated powder were analyzed with an X-ray diffractometer(XRD) operated at30kV and20mA from15to708(Rigaku,D/ MAX-B,Japan)with a wavelength of Cu K a(l¼1:5406A˚).The particle size and distribution of the precursor and spinel LiMn2O4powder were examined by laser scat-tering(Horiba,LA300,Japan).The particle morphology was observed using afield emission scanning electron microscope(FESEM,JSM-6500F,JEOL,Japan)at an accel-erating voltage of15kV.2.3.Electrochemical characterizationThe electrochemical behavior of LiMn2O4powder was examined by using two-electrode test cells which consisted of a cathode,a metallic lithium anode,a polypropylene Table1Sample designation of Li1þx Mn2O4seriesSample designation FormulationLMO LiMn2O4L1.02MO Li1.02Mn2O4L1.05MO Li1.05Mn2O4L1.08MO Li1.08Mn2O4L1.1MO Li1.1Mn2O4Fig.1.TG/DTA trace of Li1.08Mn2O4precursor heat-treated from room temperature to10008C at the rate of108C/min in air.H.W.Chan et al./Journal of Power Sources115(2003)110–118111separator and an electrolyte of 1M LiPF 6in a 1:1(volume ratio)mixture of ethylene carbonate (EC)and dimethyl carbonate (DMC).All procedures in fabricating the cells were carried out in a specially-designed chamber with low oxygen pressure and low moisture.The slurry was made of lithium manganese oxide powders with carbon black and PVDF in the weight ratio of 85:10:5.The cathodes were prepared by casting the slurry on to an aluminum foil,and then drying at 1208C for 12h.Cells were cycled within the potential range 3.0–4.2V at the 0.1C rate for the first cycle and then at the 0.2C rate for the second cycle,onwards.3.Results and discussion 3.1.Thermal analysisIn order to determine the chemical reaction of the pre-cursor and the calcining temperature of the co-precipitation products,thermal analysis is necessary to de fine the opti-mum heat-treated temperature.A TG/DTA trace of the decomposition of the precursor for a powder of Li 1.08Mn 2O 4at 108C/min in air is presented in Fig.1.The TGA curve can be divided into three regions:(i)Fig.2.XRD pattern of LiMn 2O 4precursor heat-treated at 3008C for 1h.Fig.3.XRD pattern of LiMn 2O 4precursor heat-treated at various temperatures for 10h:(a)precursor;(b)4008C;(c)5008C;(d)6008C;(e)7008C;(f)8008C.112H.W.Chan et al./Journal of Power Sources 115(2003)110–118an initial weight loss of 5%near 1008C;(ii)a further weight loss of 35%between 200and 3008C;(iii)a final weight loss of 9%that is nearly completed at 3508C.In addition,the DTA curve of Fig.1shows an endothermic peak around 1008C,which is assigned to the evaporation of free water which has been absorbed from the atmosphere.The endothermic peak around 2508C can be attributed to hydra-tion in the molecules of Li 2O 2and Mn 3O 4,which was con firmed by XRD,as shown in Fig.2.The two large exothermic peaks in the final temperature range between 290and 3308C might be due to the decomposition of the residual organics in Li 2O 2and Mn 3O 4that then form the spinel phase,LiMn 2O 4.From the TG/DTA trace,the total weight loss of the Li 1þx Mn 2O 4precursor is about 50%.Therefore,the lowest temperature of Li 1þx Mn 2O 4to form the spinel structure is taken to be 3808C.3.2.Phase identificationIn order to con firm the phase composition and the crystal-linity of the heat-treated powder,analysis by XRD and EPMA is essential.The co-precipitated precursor was heated at elevated temperatures for its evolution to the final spinel structure.The XRD pattern of the precursor LiMn 2O 4is showninFig.4.XRD pattern of (a)LiMn 2O 4and (b)Li 1.08Mn 2O 4precursor heat-treated at elevated temperature for 15h.H.W.Chan et al./Journal of Power Sources 115(2003)110–118113Fig.3a .The data indicate that the as-fabricated precursor is amorphous without crystallinity.At 4008C for 10h,there appears some broad diffraction peaks of low intensity,which indicate the incomplete formation of LiMn 2O 4.Several minor peaks are also present,which are identi fied as Mn 2O 3,as shown in Fig.3b .With increase in temperature,the peak intensity and crystallinity of LiMn 2O 4increases,while the impurity phase Mn 2O 3disappears.Lithium man-ganese oxide with well-developed crystallinity is obtained at 8008C for 10h (see Fig.3f ).Although the impurity phase Mn 2O 3is observed in the initial stage,increasing the heat-treated time may be a good way to solve this problem.LiMn 2O 4was further calcined at various temperatures for 15h as shown in Fig.4.No impurity phase is observed from 400to 8708C and a high crystalline spinel phase at high temperature can be obtained.Thus,it is concluded that an effective way to avoid the formation of the impurity phase of Mn 2O 3is by raising the heat-treated temperature or by prolonging the sintering time.The broadening of diffraction peaks at high scattered angles is indicative of residual strains [11],which are caused by inhomogeneities,cation or anion non-stoichiometry vacancies,and grain boundary effects in the structure.In fact,the strains can be reduced or removed through an increase in the heat-treatment temperature.The presence of a LiMn 2O 4major phase with impurity Mn 2O 3can be detected from a back-scattered electron image (BEI)of the morphology which is obtained by EPMA.For the precursor LiMn 2O 4calcined at 7008C for 5h,the impurity phase Mn 2O 3is observed on the basis of the XRD pattern,as indicated in Fig.5a .The white aggregation within the grey matrix of the BEI morphology in Fig.5b corresponds to Mn 2O 3.After a further 5h of heat-treatment,i.e.for a total period of 10h,the white aggregation dis-appears and only a grey matrix is observed.This indicates a more homogeneous phase,as shown in Fig.5c .Quantitative analysis by EPMA shows that the Mn:O ratio in the white aggregate in Fig.5b is 2:3,while that in Fig.5c is 2:4.This provides further evidence that the impurity phase for short-time sintering is Mn 2O 3,but LiMn 2O 4prevails after a long period oftime.Fig.5.XRD pattern of (a)LiMn 2O 4calcined at 7008C for 5h and BEI morphology of LiMn 2O 4calcined at 7008C for (b)5h and (c)10h.Table 2Phase distribution for Li 1þx Mn 2O 4sintered at various temperatures for 5–15h Phaseidentification Time (h)Temperature (8C)700750800850870LMO5MP a MSP b MSP MP MP 10PSP c PSP PSP PSP PSP 15PSP PSP PSP PSP PSP L 1.02MO5MP MP MSP MSP MSP 10PSP PSP PSP PSP PSP 15PSP PSP PSP PSP PSP L 1.05MO5MSP MP PSP MSP MSP 10PSP PSP PSP PSP PSP 15PSP PSP PSP PSP PSP L 1.08MO5PSP PSP MP MSP PSP 10PSP PSP PSP PSP PSP 15PSP PSP PSP PSP PSP L 1.1MO5MSP MSP MSP PSP PSP 10PSP PSP PSP PSP PSP 15PSPPSPPSPPSPPSPaMP:mixed phase of spinel and Mn 2O 3.bMSP:major spinel phase with trace Mn 2O 3peak visible.cPSP:pure spinel phase without other detectable phase.114H.W.Chan et al./Journal of Power Sources 115(2003)110–118ttice constant vs.heat-treated temperature for calcined LiMn 2O 4for 10h.Fig.7.FESEM images of various powders calcined at 8708C for 15h:(a)LiMn 2O 4;(b)Li 1.08Mn 2O 4;(c)Li 1.1Mn 2O 4.H.W.Chan et al./Journal of Power Sources 115(2003)110–118115Precursors with nominal LiMn 2O 4and Li excess Li 1þx Mn 2O 4were sintered at various temperatures for dif-ferent periods.Detailed phase identi fications for powders sintered under various conditions have been carried out and are summarized in Table 2.It is concluded that pure spinel phase is obtained for powders sintered at high temperature for long periods.For powders sintered for only 5h at atemperature below 8008C,an impurity phase of Mn 2O 3is observed.Nearly all the Mn 2O 3disappears,however,if sintering is conducted for more than 10h.It should be noted that powders sintered as low as 7008C for more than 10h also exhibit a pure spinel phase,as indicated in Table 2.A larger particle size in the range 5–10m m is required to assemble a Li-ion battery for prac-tical applications.Thus,powders sintered at higher tem-perature,such as those shown in the upper right section of Table 2,are more suitable for battery assembly.The data in Fig.4b show that the XRD peaks shift towards higher diffraction angles.This is attributed to a small change in the crystal structure.There is also an implication of the fact that a trace amount of the excess Li þions has been indeed been doped into the cubic spinel structure.On the basis of the XRD results,the lattice constant of calcined powder was evaluated by a least-squares program,and then plotted against the calcined temperature,as indi-cated in Fig.6.The lattice constant increases with tempera-ture from 8.17A˚at 4008C to 8.26A ˚at 8508C.This behavior suggests the gradual formation of stoichiometric spinel [12].3.3.Particle size and morphologyMicrographs of sintered samples of LiMn 2O 4,Li 1.08Mn 2O 4and Li 1.1Mn 2O 4derived from the co-precipita-tion method and calcined at 8708C for 15h are shown in Fig.7a –c ,respectively.The morphology of Fig.7a –c is corresponding to that of single-crystal with a cubic structure.The particles have a well-developed octahedral structure which is bounded by eight (111)planes [13].The average particle size of LiMn 2O 4,Li 1.08Mn 2O 4and Li 1.1Mn 2O 4calcined at 8708C for 15h is 7.4,8.1,and 8.3m m,respectively,as shown in Fig.8a –c .It appears that the particle size of LiMn 2O 4is smaller than that of Li 1.08Mn 2O 4and Li 1.1Mn 2O 4.The difference in particle size is considered to be due to the change of the valence of manganese that results from Li þdoping.It is argued that excess lithium enhances the stability of the structure and allows more Li þions to intercalate or de-intercalate through the cathode and the anode.3.4.Electrochemical propertiesThe electrochemical behavior of lithium manganese oxide powders was also investigated.The first charge and discharge curves for Li/Li 1þx Mn 2O 4coin cells (x ¼0,0.08and 0.1)operated between 3and 4.2Vare presented in Fig.8.The first irreversible capacity of LiMn 2O 4is 6mAh g À1,i.e.the difference between 90mAh g À1for first charge capacity and 84mAh g À1for first discharge capacity,as shown in Fig.9a .Li 1.08Mn 2O 4exhibits a value of 135and 124mAh g À1for the charge and discharge capacities,respectively,and thus an irreversible capacity of 11mAh g À1is lost.Excess Li þions in the LiMn 2O 4powders leads to a considerablyhighFig.8.Particle-size distribution of various powders calcined at 8708C for 15h:(a)LiMn 2O 4;(b)Li 1.08Mn 2O 4;(c)Li 1.1Mn 2O 4.116H.W.Chan et al./Journal of Power Sources 115(2003)110–118initial speci fic capacity and this partially compensates for the irreversible capacity loss.The rate of capacity fading of Li 1þx Mn 2O 4(x ¼0,0.08and 0.1)was measured galvanostatically between 3and 4.2V for cells of con figuration:Li j EC þDMC þLiPF 6j Li 1þx Mn 2O 4.The capacity of LiMn 2O 4calcined at 8708C for 15h is 84.3mAh g À1for the first cycle but decays to 64.7mAh g À1after 16cycles.The discharge capacity of Li 1.08Mn 2O 4cal-cined at 8708C for 15h is 123.6mAh g À1for the initial cycle but retains 87%of its origin value after 16cycles,which is a much better performance than that exhibited by LiMn 2O 4,as shown in Fig.10.The larger decay in LiMn 2O 4is due to the tail of the charge curve at 3.2V and the plateau of the discharge curve between 4.1and 4.2V ,which is caused by phase transformation during charging and discharging,asshown in Fig.9a .The findings in this study demonstrate that cathode materials composed of excess Li spinel lithium manganese oxide and prepared by the co-precipitation method show relatively good stability and high speci fic capacity.4.ConclusionsThe following observations are made.1.Li 1þx Mn 2O 4powders doped with excess lithium are derived by the co-precipitation method with LiOH as the reactant,and sintered at temperatures between 400and 8708C for 5–15h.Fig.9.First charge and discharge curves of various powders calcined at 8708C for 15h at the 0.1C rate (a)LiMn 2O 4and (b)Li 1.08Mn 2O 4.H.W.Chan et al./Journal of Power Sources 115(2003)110–1181172.With increase in sintering time and temperature,the initially formed Mn 2O 3impurity is removed and a fully spinel structure is obtained.3.The particle size of LiMn 2O 4and excess Li doped LiMn 2O 4calcined at 8708C for 15h is in the range 7–8m m,which is very suitable for fabrication of coin cells.4.Spinel Li 1þx Mn 2O 4(x ¼0:08and 0.1)with uniform particle-size distribution shows good intercalation and de-intercalation performance and high initial capacity.AcknowledgementsThe first two authors are grateful to the Coremax Taiwan Corporation,Taiwan,for the financial support,with special thanks to the General Manager Mr.Jim Ho.The technical assistance by Mr.S.D.Wu in cell assembly is also appre-ciated.Partial support from National Science Council under the Contract No.NSC-90-2216-E007-070is acknowledged.References[1]G.T.K.Fey,K.S.Wang,S.M.Yang,J.Power Sources 68(1997)159.[2] D.G.Wickham,W.J.Croft,J.Phys.Chem.Sloids 7(1958)351.[3]J.C.Hunter,J.Solid State Chem.39(1981)142.[4]M.M.Thackeray,W.I.F.David,P.G.Bruce,J.B.Goodenough,Mater.Res.Bull.18(1983)461.[5]R.T.Cygan,H.R.Westrich,D.H.Doughty,Mater.Res.Symp.Proc.393(1995)113.[6]J.M.Tarascan, F.Coowar,G.Amatuci, F.K.Shokoohi, D.G.Guyomard,J.Power Sources 54(1995)103.[7]R.Manev,A.Momchilv,A.Nassalevska,A.Sato,J.Power Sources 54(1995)323.[8]Q.Zhong,U.Sachen,J.Power Sources 54(1995)221.[9]J.M.Tarascan,D.G.Guyomard,J.Electrochem.Soc.138(1991)2864.[10]R.J.Gummow,M.M.Thackeray,J.Electrochem.Soc.141(1994)1178.[11]R.Koksbang,J.Barker,H.Shi,M.Y .Saidi,Solid State Ionics 89(1996)1.[12] A.R.Naghash,J.Y .Lee,J.Power Sources 85(2000)284.[13]Y .K.Sun,B.Oh,H.J.Lee,Electrochim.Acta 46(2001)541.Fig.10.Specific discharge capacities of Li 1þx Mn 2O 4calcined at 8708C for 15h cycled at the 0.2C rate at room temperature.118H.W.Chan et al./Journal of Power Sources 115(2003)110–118。