Fluent6.2燃料电池帮助手册
ANSYS Fluent 15.0 燃料电池模块手册(en)
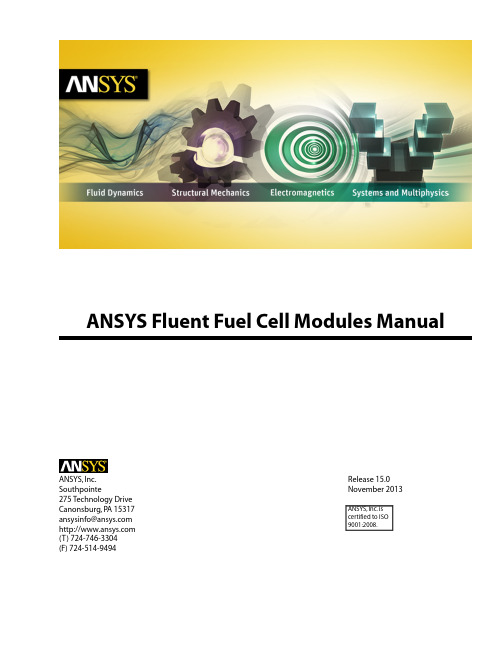
Disclaimer Notice
THIS ANSYS SOFTWARE PRODUCT AND PROGRAM DOCUMENTATION INCLUDE TRADE SECRETS AND ARE CONFIDENTIAL AND PROPRIETARY PRODUCTS OF ANSYS, INC., ITS SUBSIDIARIES, OR LICENSORS. The software products and documentation are furnished by ANSYS, Inc., its subsidiaries, or affiliates under a software license agreement that contains provisions concerning non-disclosure, copying, length and nature of use, compliance with exporting laws, warranties, disclaimers, limitations of liability, and remedies, and other provisions. The software products and documentation may be used, disclosed, transferred, or copied only in accordance with the terms and conditions of that software license agreement. ANSYS, Inc. is certified to ISO 9001:2008.
ANSYS Fluent Fuel Cell Modules Manual
Fluent-v6.2-lect-06-turbulence
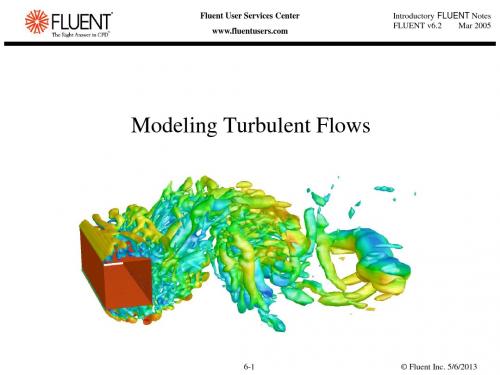
u(x, t ) = u (x, t ) + u′(x, t )
resolved scale subgrid scale
ui ν x j
Filter;
Sub-grid scale (SGS) turbulent stress
Filtered N-S equation
ui ui u j 1 p + = + ρ xi x j t x j
6-2 Fluent Inc. 9/7/2010
Fluent User Services Center
Introductory FLUENT Notes FLUENT v6.2 Mar 2005
Is the Flow Turbulent?
External Flows
Fluent User Services Center
Introductory FLUENT Notes FLUENT v6.2 Mar 2005
Modeling Turb2010
Fluent User Services Center
ui τ ij ν x x j j
τ ij ≡ ui u j ui u j
Spectrum of turbulent eddies in the Navier-Stokes equations is filtered:
The filter is a function of grid size Eddies smaller than the grid size are removed and modeled by a sub-grid scale (SGS) model Larger eddies are directly solved numerically by the filtered transient N-S equation
Fluent使用指南
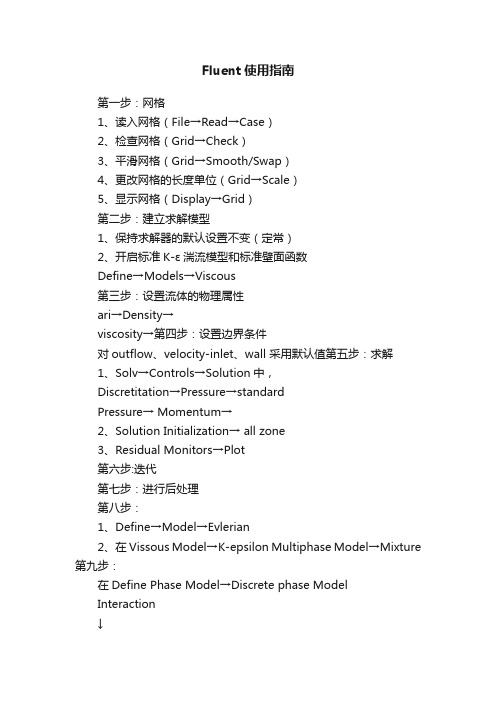
Fluent使用指南第一步:网格1、读入网格(File→Read→Case)2、检查网格(Grid→Check)3、平滑网格(Grid→Smooth/Swap)4、更改网格的长度单位(Grid→Scale)5、显示网格(Display→Grid)第二步:建立求解模型1、保持求解器的默认设置不变(定常)2、开启标准K-ε湍流模型和标准壁面函数Define→Models→Viscous第三步:设置流体的物理属性ari→Density→viscosity→第四步:设置边界条件对outflow、velocity-inlet、wall 采用默认值第五步:求解1、Solv→Controls→Solution中,Discretitation→Pressure→standardPressure→ Momentum→2、Solution Initialization→ all zone3、Residual Monitors→Plot第六步:迭代第七步:进行后处理第八步:1、Define→Model→Evlerian2、在Vissous Model→K-epsilon Multiphase Model→Mixture 第九步:在Define Phase Model→Discrete phase ModelInteraction↓选中→Interaction With Continuous Phase Nomber of Continuous PhaseInteractions per DPM Interaction第十步:设置物理属性第十一步:Define→Operating →重力加速度Define→Boondary Conditionsflvid→Mixture→选中Sovrce Terms 其他默认Phase-1→选中Sovrce Terms 其他默认Phase-2→选中Sovrce Terms 其他默认inflow→Mixture→全部默认Phase-1→全部默认Phase-2→Multiphase→Volume Fraction→其他默认outflow→Mixture→默认Phase-1→默认Phase-2→默认wall→Mixture→全部默认Phase-1→默认Phase-2默认第十二步:Slove→Controls→Slution Controls→Pressure→ Momentum→其余默认第十三步:千万不能再使用初始化第十四步:进行迭代计算截Z轴上的图:在Surface→iso↓Surface of constant↓Grid↓然后选x、y、z轴(根据具体情况而定)↓在Iso-Values→选取位置C的设置在New Surface Name中输入新各字→点创建然后在Display→Grid→Edge type→Feature→选中刚创建的那个面,然后Display查看刚才那面是否创建对最后在Display→Contours→Options→Filled→Surface→选中面,然后Display。
Fluent辅导指南
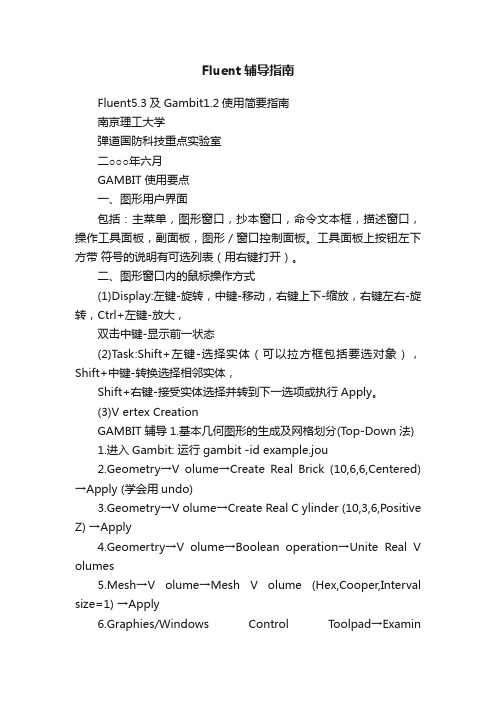
Fluent辅导指南Fluent5.3及Gambit1.2使用简要指南南京理工大学弹道国防科技重点实验室二○○○年六月GAMBIT使用要点一、图形用户界面包括:主菜单,图形窗口,抄本窗口,命令文本框,描述窗口,操作工具面板,副面板,图形/窗口控制面板。
工具面板上按钮左下方带符号的说明有可选列表(用右键打开)。
二、图形窗口内的鼠标操作方式(1)Display:左键-旋转,中键-移动,右键上下-缩放,右键左右-旋转,Ctrl+左键-放大,双击中键-显示前一状态(2)Task:Shift+左键-选择实体(可以拉方框包括要选对象),Shift+中键-转换选择相邻实体,Shift+右键-接受实体选择并转到下一选项或执行Apply。
(3)V ertex CreationGAMBIT辅导1.基本几何图形的生成及网格划分(Top-Down法)1.进入Gambit: 运行gambit -id example.jou2.Geometry→V olume→Create Real Brick (10,6,6,Centered) →Apply (学会用undo)3.Geometry→V olume→Create Real C ylinder (10,3,6,Positive Z) →Apply4.Geomertry→V olume→Boolean operation→Unite Real V olumes5.Mesh→V olume→Mesh V olume (Hex,Cooper,Interval size=1) →Apply6.Graphies/Windows Control Toolpad→ExaminMesh(Range,EquiAngle Skew)7.File→ExitGAMBIT辅导2.二维混合弯管(Bottom-Up法)1.选择Solver→Fluent42.T ools→Coordinate System→Display Grid(Visibility,XY,X,-32,32,16)→Update list→(Y,-32,32,16) →Update list→Snap→Apply3.用Ctrl+右键生成九个点(A→H)4.取消Display Grid 面板中的Visibility选项→Apply5.Geometry→Edge→Create EDGE→Create Real Circular Arc(中心E,边FD)→Apply Geometry→Edge→Create EDGE→Create Real Circular Arc(中心E,边GB)→Apply6.Geometry→Edge→Create Edge→Create Straight Edge(B→A,A→C,C→D,F→G,G→I,I→H,H→F)7.Geometry→Edge→Split/Edge (GB,Type=Cylinder,local t=-39.93) →Apply (生成J点)G eometry→Edge→Split Edge(JB,Type=Cylinder,local t=-50.07) →Apply (生成K点)G eometry→V ertex→More/Copy vertices (K 点,Copy,Translate,x=0,y=-12.0,z=1)→Apply (生成L点)G eometry→V ertex→More/Copy V ertices (L 点,Copy,Translate,x=4,y=0,z=0) →Apply (生成M点) 8.Geometry→Edge→Create Edge→Create Straight Edge(K→L, L→M,M→J)9.Geomety→Face→Create Face→Create Face From Edge(KJ,JG,GI,IH,HF,FD,DC,CA,AB,BK)→ApplyGeomety→Face→Create Face→Create Face From Edge (KJ,JM,ML,Lk)→Apply10.Mesh→Edge→Mesh Edge (AC,HI,Ratio=1.25,DoubleSided, Interval Count=10) →Apply Mesh→Edge→Mesh Edge (AB,CD,GI,FH, Interval Count=15)→ApplyMesh→Edge→Mesh Edge (BK,JG,Interval Count=12,,Ratio=0.9)→ApplyMesh→Edge→Mesh Ed ge(KJ,Ratio0.85,Double Sided,不选Spacing Apply,不选Option-Mesh项)-Apply 11.Mesh→Face→Mesh Faces(大面,Quad,Map,Inteval size=1)→ApplyMesh→Face→Mesh Faces(小面,Quad,Map,Inteval size=1)→Apply12.Zones→Specify Boundy Type (inflow1,INFLOW,Entity=Edges,AC边,INFLOW,LM边,outflow, OUTFLOW,HI边) →Apply 注:Zones→Specify Continuum Types 默认为流体,其余面默认为墙。
(完整版)《FLUENT中文手册(简化版)》
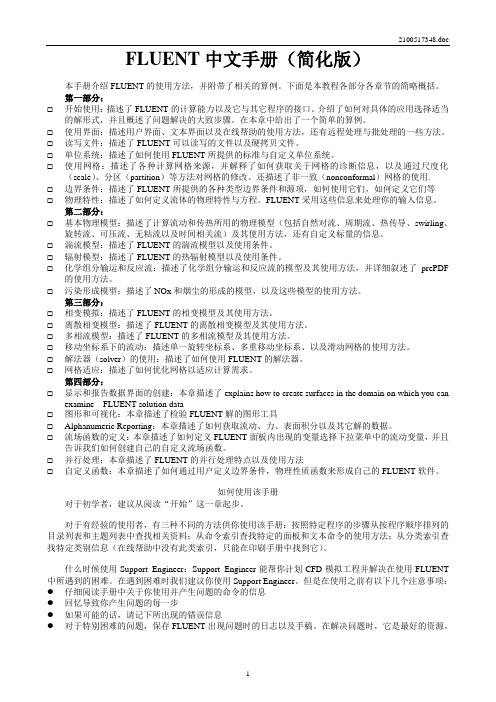
FLUENT中文手册(简化版)本手册介绍FLUENT的使用方法,并附带了相关的算例。
下面是本教程各部分各章节的简略概括。
第一部分:☐开始使用:描述了FLUENT的计算能力以及它与其它程序的接口。
介绍了如何对具体的应用选择适当的解形式,并且概述了问题解决的大致步骤。
在本章中给出了一个简单的算例。
☐使用界面:描述用户界面、文本界面以及在线帮助的使用方法,还有远程处理与批处理的一些方法。
☐读写文件:描述了FLUENT可以读写的文件以及硬拷贝文件。
☐单位系统:描述了如何使用FLUENT所提供的标准与自定义单位系统。
☐使用网格:描述了各种计算网格来源,并解释了如何获取关于网格的诊断信息,以及通过尺度化(scale)、分区(partition)等方法对网格的修改。
还描述了非一致(nonconformal)网格的使用.☐边界条件:描述了FLUENT所提供的各种类型边界条件和源项,如何使用它们,如何定义它们等☐物理特性:描述了如何定义流体的物理特性与方程。
FLUENT采用这些信息来处理你的输入信息。
第二部分:☐基本物理模型:描述了计算流动和传热所用的物理模型(包括自然对流、周期流、热传导、swirling、旋转流、可压流、无粘流以及时间相关流)及其使用方法,还有自定义标量的信息。
☐湍流模型:描述了FLUENT的湍流模型以及使用条件。
☐辐射模型:描述了FLUENT的热辐射模型以及使用条件。
☐化学组分输运和反应流:描述了化学组分输运和反应流的模型及其使用方法,并详细叙述了prePDF 的使用方法。
☐污染形成模型:描述了NOx和烟尘的形成的模型,以及这些模型的使用方法。
第三部分:☐相变模拟:描述了FLUENT的相变模型及其使用方法。
☐离散相变模型:描述了FLUENT的离散相变模型及其使用方法。
☐多相流模型:描述了FLUENT的多相流模型及其使用方法。
☐移动坐标系下的流动:描述单一旋转坐标系、多重移动坐标系、以及滑动网格的使用方法。
Fluent-v6.2-lect-08-udfs
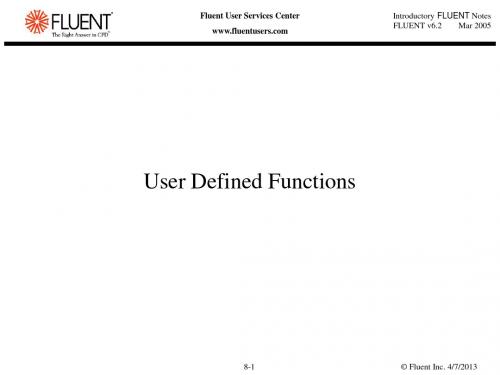
y=0
8-6
© Fluent Inc. 5/16/2012
Fluent User Services Center
Introductory FLUENT Notes FLUENT v6.2 Mar 2005
Step 1: Source Code
The DEFINE_PROFILE macro allows the function inlet_x_velocity to be defined.
Why build UDF’s?
Standard interface cannot be programmed to anticipate all needs.
Customization of boundary conditions, source terms, reaction rates, material properties, etc. Adjust functions (once per iteration) Execute on Demand functions Solution Initialization
Fluent User Services Center
Introductory FLUENT Notes FLUENT v6.2 Mar 2005
User Defined Functions
8-1
© Fluent Inc. 5/16/2012
Fluent User Services Center
8-4
© Fluent Inc. 5/16/2012
Fluent User Services Center
Introductory FLUENT Notes FLUENT v6.2 Mar 2005
Fluent-v6.2.02
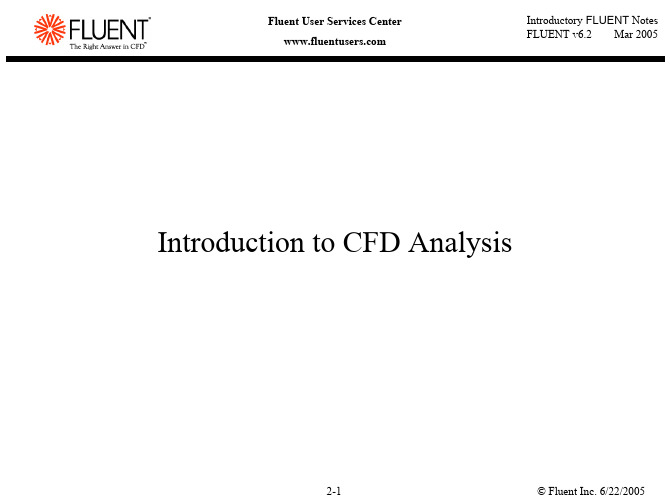
Fluid region of pipe flow is discretized into a finite set of control volumes (mesh).
∂ ∫ ρφdV + ∫ ρφV ⋅ dA = ∫ Γ∇φ ⋅ dA + V Sφ dV ∫ ∂t V A A
unsteady convection diffusion generation
User-defined functions (written in C) in FLUENT 6 User-defined subroutines (written in FORTRAN) in FLUENT 4.5
What degree of accuracy is required? How quickly do you need the results?
Problem Identification and Pre-Processing 1. Define your modeling goals. 2. Identify the domain you will model. 3. Design and create the grid
Gas Cyclone Riser
tetrahedron
hexahedron
Do you have sufficient computer memory?
How many cells are required? How many models will be used?
pyramid
prism/wedge
2-8 © Fluent Inc. 6/22/2005
Fluent User Services Center
Introductory FLUENT Notes FLUENT v6.2 Mar 2005
海川化工论坛_Fluent帮助文件
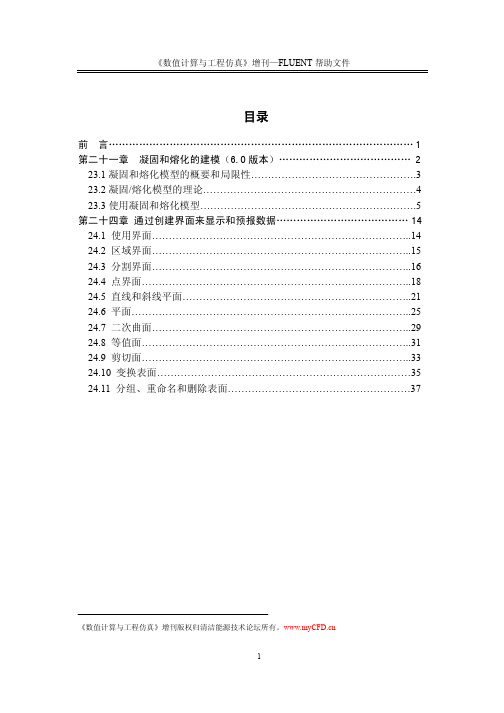
目录前 言 (1)第二十一章 凝固和熔化的建模(6.0版本) (2)23.1凝固和熔化模型的概要和局限性 (3)23.2凝固/熔化模型的理论 (4)23.3使用凝固和熔化模型 (5)第二十四章 通过创建界面来显示和预报数据 (14)24.1 使用界面 (14)24.2 区域界面 (15)24.3 分割界面 (16)24.4 点界面 (18)24.5 直线和斜线平面 (21)24.6 平面 (25)24.7 二次曲面 (29)24.8 等值面 (31)24.9 剪切面 (33)24.10 变换表面 (35)24.11 分组、重命名和删除表面 (37)《数值计算与工程仿真》增刊版权归清洁能源技术论坛所有。
前言本次翻译工作是由清洁能源技术论坛的会员“过滤与分离者”于2004年11月提出的,经过几个月的翻译整理,最终汇集成稿,当然由于作者水平有限,在翻译中还存在不少的问题,希望大家批评指正,以便我们进一步改进。
在翻译期间,得到“清洁能源技术论坛”各位会员的大力支持,具体的翻译工作如下:wangujunli21章第一、二节1-8页bruce21章第三节9-14页summered 24章第一至三节1-7页jordanupc 24章第四至五节8-15页(后由vvvms代替完成)jdaa0524 24章第六至七节 16-23页(后由xunbao 代替完成)xiongbin24章第八至九节 24-29页xamaomm 24章第十至十一节 29-34页本次工作由jackywzq、bitzhangjie、caohuali和sfsm编辑整理完成,本次工作还得到了“清洁能源技术论坛”论坛的brightsun、caoqx、gaojm等几位版主的大力支持,在此对他们付出的心血和汗水表示衷心感谢。
(说明:第二十一章的前两节是依据FLUENT6.1版本翻译完成的,其余的章节是依据FLUENT6.0版本翻译完成的)清洁能源技术论坛《数值计算与工程仿真》增刊版权归清洁能源技术论坛所有。
Fluent-v6.2-lect-05-solversettings

Introductory FLUENT Notes FLUENT v6.2 Mar 2005
Outline
Using the Solver Setting Solver Parameters Convergence Definition Monitoring Stability Accelerating Convergence Accuracy Grid Independence Grid Adaption Unsteady Flows Modeling Unsteady-flow problem setup Non-iterative Transient Advancement (NITA) schemes Unsteady flow modeling options Summary Appendix
Introductory FLUENT Notes FLUENT v6.2 Mar 2005
Choosing a Solver
Choices are Coupled-Implicit, Coupled-Explicit, or Segregated (Implicit) The coupled solvers are recommended if a strong inter-dependence exists between density, energy, momentum, and/or species e.g., high speed compressible flow or finite-rate reaction flows In general, the coupled-implicit solver is recommended over the coupled-explicit solver Time required: Implicit solver runs roughly twice as fast Memory required: Implicit solver requires roughly twice as much memory as coupledexplicit or segregated solvers! Improved pre-conditioning in Fluent v6.2 for the coupled-implicit solver enhances accuracy and robustness for low-Mach number flows The coupled-explicit solver should only be used for unsteady flows when the characteristic time scale of problem is on same order as that of the acoustics e.g., tracking transient shock wave The segregated (implicit) solver is preferred in all other cases. Lower memory requirements than coupled-implicit solver Segregated approach provides flexibility in solution procedure
Fluent-v6.2-lect-01-intro
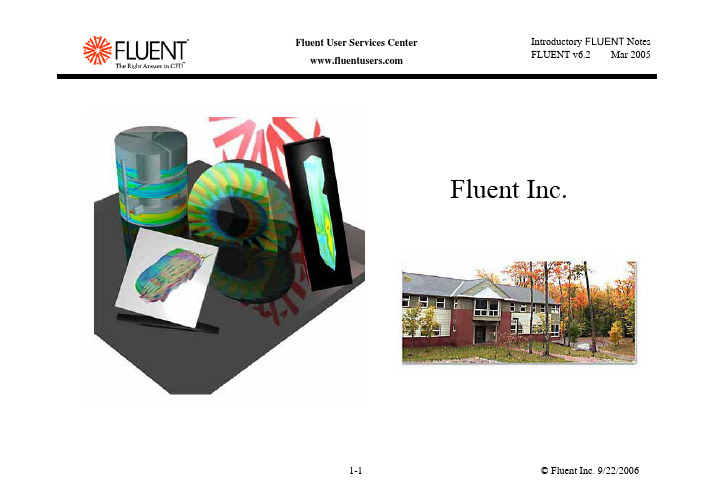
/andFLUENT 6.2Flow-induced noise predictionSurface pressuredistribution in anautomotive engine Instantaneous solids concentration in a riser indicating uniform distribution of catalyst at the riser head.rigid wallrigidwallcompliantwallinitial mesh刚性动脉变形动脉Time history plot of wall shear rate-Deformations cannot be neglected!Velocity contour plotInverse Die Design:Determines die geometrybased upon desiredextruded shape.Requested part shape and calculated dielip shape for a rubber car door seal. flows (“memoryFluent User Services Center Introductory FLUENT Notes FLUENT v6.2 Mar 2005IcePakIcePak is focused on electronicscooling design:Cooling airflow, heat conduction, convection and radiation heat transferThe user interface and automatic meshing are tailored for applications such as:Cabinet design Fan placement Board-level design Heat sink evaluation Flow pathlines and temperature distribution in a fan-cooled computer cabinet.1-11© Fluent Inc. 9/22/2006Fluent User Services Center Introductory FLUENT Notes FLUENT v6.2 Mar 2005AirpakSimplifies the design and analysis of ventilation systems Accurate, quick, and easy-to-use design tool that empowers designers and professionals, without extensive backgrounds in computer applications, to utilize the powers of advanced CFD tools Optimize your designs or pinpoint problems based on accurate predictions of airflow patterns, thermal conditions, comfort conditions, and/or contamination control effectiveness1-12© Fluent Inc. 9/22/2006Fluent User Services Center Introductory FLUENT Notes FLUENT v6.2 Mar 2005MixSimMixSim is a specialized user interface that allows quick and easy set-upof mixing tank simulations. The tank size, bottom shape, baffle configuration, number and type of impellers, etc. are specified directly. The mesh and complete problem definition are then automatically created. Other features include:Impeller libraries from leading equipment manufacturers Transient sliding mesh, steady-state multiple reference frame models 非牛顿流体流变学1-13© Fluent Inc. 9/22/2006Fluent User Services Center Introductory FLUENT Notes FLUENT v6.2 Mar 2005FloWizardOur first general purpose CFD product for non-specialists driven by FLUENT and Gambit . FloWizard’s focus is on ease of use and automation:It is a highly-automated, “first pass” simulation tool for use in basic flow and heat transfer calculations. A Wizard-based interface guides the user through all the steps of a CFD analysis, from problem set-up to postprocessing. The user is insulated from specialized CFD parameters, such as discretization and turbulence model choices.1-14© Fluent Inc. 9/22/2006Fluent User Services Center Introductory FLUENT Notes FLUENT v6.2 Mar 2005Pre-processor: GambitA single, integrated pre-processor for CFD analysis.Geometry creation Mesh generation Mesh quality examination Boundary zone assignment1-15© Fluent Inc. 9/22/2006Fluent User Services Center Introductory FLUENT Notes FLUENT v6.2 Mar 2005Pre-processor: TGridA pre-processor for tet/hybrid mesh generation. Useful when starting with triangular surface mesh.1-16© Fluent Inc. 9/22/2006Fluent User Services Center Introductory FLUENT Notes FLUENT v6.2 Mar 2005DocumentationDocumentation for all products available at Separate CD for each product (e.g., FLUENT 6, TGrid, etc.) containing all the manuals for that product. Two formats available:HTMLfor general viewing, searching, limited printingAdobe Acrobat PDFfor high quality printing of one or many pagesFluent 6.2 Documentation Web Page at 1-17 © Fluent Inc. 9/22/2006Fluent User Services Center Introductory FLUENT Notes FLUENT v6.2 Mar 2005Training EvaluationEvaluation forms handed out with training materials. Your feedback helps us improve our training materials and methods. Please turn-in your evaluations forms on Thursday before you leave.1-18© Fluent Inc. 9/22/2006。
Fluent用户手册

The FLUENT User's Guide tells you what you need to know to use FLUENT. At the end of the User's Guide, you will find a Reference Guide, a nomenclature list, a bibliography, and an index.!! Under U.S. and international copyright law, Fluent is unable to distribute copies of the papers listed in the bibliography, other than those published internally by Fluent. Please use your library or a document delivery service to obtain copies of copyrighted papers.A brief description of what's in each chapter follows:•Chapter 1, Getting Started, describes the capabilities of FLUENT and the way in which it interacts with other Fluent Inc. and third-party programs. It also advises you on how to choose the appropriate solverformulation for your application, gives an overview of the problem setup steps, and presents a samplesession that you can work through at your own pace. Finally, this chapter provides information aboutaccessing the FLUENT manuals on CD-ROM or in the installation area.•Chapter 2, User Interface, describes the mechanics of using the graphical user interface, the text interface, and the on-line help. It also provides instructions for remote and batch execution. (See the separate Text Command List for information about specific text interface commands.)•Chapter 3, Reading and Writing Files, contains information about the files that FLUENT can read and write, including hardcopy files.•Chapter 4, Unit Systems, describes how to use the standard and custom unit systems available in FLUENT.•Chapter 5, Reading and Manipulating Grids, describes the various sources of computational grids and explains how to obtain diagnostic information about the grid and how to modify it by scaling, translating, and other methods. This chapter also contains information about the use of non-conformal grids.•Chapter 6, Boundary Conditions, explains the different types of boundary conditions available in FLUENT, when to use them, how to define them, and how to define boundary profiles and volumetric sources and fix the value of a variable in a particular region. It also contains information about porousmedia and lumped parameter models.•Chapter 7, Physical Properties, explains how to define the physical properties of materials and the equations that FLUENT uses to compute the properties from the information that you input.•Chapter 8, Modeling Basic Fluid Flow, describes the governing equations and physical models used by FLUENT to compute fluid flow (including periodic flow, swirling and rotating flows, compressibleflows, and inviscid flows), as well as the inputs you need to provide to use these models.•Chapter 9, Modeling Flows in Moving Zones, describes the use of single rotating reference frames, multiple moving reference frames, mixing planes, and sliding meshes in FLUENT.•Chapter 10, Modeling Turbulence, describes FLUENT's models for turbulent flow and when and how to use them.•Chapter 11, Modeling Heat Transfer, describes the physical models used by FLUENT to compute heat transfer (including convective and conductive heat transfer, natural convection, radiative heat transfer,and periodic heat transfer), as well as the inputs you need to provide to use these models.•Chapter 12, Introduction to Modeling Species Transport and Reacting Flows, provides an overview of the models available in FLUENT for species transport and reactions, as well as guidelines for selectingan appropriate model for your application.•Chapter 13, Modeling Species Transport and Finite-Rate Chemistry, describes the finite-rate chemistry models in FLUENT and how to use them. This chapter also provides information about modeling species transport in non-reacting flows.•Chapter 14, Modeling Non-Premixed Combustion, describes the non-premixed combustion model and how to use it. This chapter includes details about using prePDF.•Chapter 15, Modeling Premixed Combustion, describes the premixed combustion model and how to use it.•Chapter 16, Modeling Partially Premixed Combustion, describes the partially premixed combustion model and how to use it.•Chapter 17, Modeling Pollutant Formation, describes the models for the formation of NOx and soot and how to use them.•Chapter 18, Introduction to Modeling Multiphase Flows, provides an overview of the models for multiphase flow (including the discrete phase, VOF, mixture, and Eulerian models), as well as guidelines for selecting an appropriate model for your application.•Chapter 19, Discrete Phase Models, describes the discrete phase models available in FLUENT and how to use them.•Chapter 20, General Multiphase Models, describes the general multiphase models available in FLUENT (VOF, mixture, and Eulerian) and how to use them.•Chapter 21, Modeling Solidification and Melting, describes FLUENT's model for solidification and melting and how to use it.•Chapter 22, Using the Solver, describes the FLUENT solvers and how to use them.•Chapter 23, Grid Adaption, explains the solution-adaptive mesh refinement feature in FLUENT and how to use it.•Chapter 24, Creating Surfaces for Displaying and Reporting Data, explains how to create surfaces in the domain on which you can examine FLUENT solution data.•Chapter 25, Graphics and Visualization, describes the graphics tools that you can use to examine your FLUENT solution.•Chapter 26, Alphanumeric Reporting, describes how to obtain reports of fluxes, forces, surface integrals, and other solution data.•Chapter 27, Field Function Definitions, defines the flow variables that appear in the variable selection drop-down lists in FLUENT panels, and tells you how to create your own custom field functions. •Chapter 28, Parallel Processing, explains the parallel processing features in FLUENT and how to use them. This chapter also provides information about partitioning your grid for parallel processing.18. Introduction to Modeling Multiphase FlowsA large number of flows encountered in nature and technology are a mixture of phases. Physical phases of matter are gas, liquid, and solid, but the concept of phase in a multiphase flow system is applied in a broader sense. In multiphase flow, a phase can be defined as an identifiable class of material that has a particular inertial response to and interaction with the flow and the potential field in which it is immersed. For example, different-sized solid particles of the same material can be treated as different phases because each collection of particles with the same size will have a similar dynamical response to the flow field.This chapter provides an overview of multiphase modeling in FLUENT, and Chapters 19 and 20 provide details about the multiphase models mentioned here. Chapter 21 provides information about melting and solidification.18.1 Multiphase Flow RegimesMultiphase flow can be classified by the following regimes, grouped into four categories:gas-liquid or liquid-liquid flowsbubbly flow: discrete gaseous or fluid bubbles in a continuous fluiddroplet flow: discrete fluid droplets in a continuous gasslug flow: large bubbles in a continuous fluidstratified/free-surface flow: immiscible fluids separated by a clearly-defined interfacegas-solid flowsparticle-laden flow: discrete solid particles in a continuous gaspneumatic transport: flow pattern depends on factors such as solid loading, Reynolds numbers, and particle properties. Typical patterns are dune flow, slug flow, packed beds, and homogeneous flow.fluidized beds: consist of a vertical cylinder containing particles where gas is introduced through a distributor. The gas rising through the bed suspends the particles. Depending on the gas flow rate, bubbles appear and rise through the bed, intensifying the mixing within the bed.liquid-solid flowsslurry flow: transport of particles in liquids. The fundamental behavior of liquid-solid flows varies with the properties of the solid particles relative to those of the liquid. In slurry flows, the Stokes number (seeEquation 18.4-4) is normally less than 1. When the Stokes number is larger than 1, the characteristic of the flow is liquid-solid fluidization.hydrotransport: densely-distributed solid particles in a continuous liquidsedimentation: a tall column initially containing a uniform dispersed mixture of particles. At the bottom, the particles will slow down and form a sludge layer. At the top, a clear interface will appear, and in the middle a constant settling zone will exist.three-phase flows (combinations of the others listed above)Each of these flow regimes is illustrated in Figure 18.1.1.Figure 18.1.1: Multiphase Flow Regimes18.2 Examples of Multiphase SystemsSpecific examples of each regime described in Section 18.1 are listed below:Bubbly flow examples: absorbers, aeration, air lift pumps, cavitation, evaporators, flotation, scrubbersDroplet flow examples: absorbers, atomizers, combustors, cryogenic pumping, dryers, evaporation, gas cooling, scrubbersSlug flow examples: large bubble motion in pipes or tanksStratified/free-surface flow examples: sloshing in offshore separator devices, boiling and condensation in nuclear reactorsParticle-laden flow examples: cyclone separators, air classifiers, dust collectors, and dust-laden environmental flowsPneumatic transport examples: transport of cement, grains, and metal powdersFluidized bed examples: fluidized bed reactors, circulating fluidized bedsSlurry flow examples: slurry transport, mineral processingHydrotransport examples: mineral processing, biomedical and physiochemical fluid systemsSedimentation examples: mineral processing18.3 Approaches to Multiphase ModelingAdvances in computational fluid mechanics have provided the basis for further insight into the dynamics of multiphase flows. Currently there are two approaches for the numerical calculation of multiphase flows: the Euler-Lagrange approach and the Euler-Euler approach.18.3.1 The Euler-Lagrange ApproachThe Lagrangian discrete phase model in FLUENT (described in Chapter 19) follows the Euler-Lagrange approach. The fluid phase is treated as a continuum by solving the time-averaged Navier-Stokes equations, while the dispersed phase is solved by tracking a large number of particles, bubbles, or droplets through the calculated flow field. The dispersed phase can exchange momentum, mass, and energy with the fluid phase.A fundamental assumption made in this model is that the dispersed second phase occupies a low volume fraction, even though high mass loading ( ) is acceptable. The particle or droplet trajectories are computed individually at specified intervals during the fluid phase calculation. This makes the model appropriate for the modeling of spray dryers, coal and liquid fuel combustion, and some particle-laden flows, but inappropriate for the modeling of liquid-liquid mixtures, fluidized beds, or any application where the volume fraction of the second phase is not negligible.18.3.2 The Euler-Euler ApproachIn the Euler-Euler approach, the different phases are treated mathematically as interpenetrating continua. Since the volume of a phase cannot be occupied by the other phases, the concept of phasic volume fraction is introduced. These volume fractions are assumed to be continuous functions of space and time and their sum is equal to one. Conservation equations for each phase are derived to obtain a set of equations, which have similar structure for all phases. These equations are closed by providing constitutive relations that are obtained from empirical information, or, in the case of granular flows , by application of kinetic theory.In FLUENT, three different Euler-Euler multiphase models are available: the volume of fluid (VOF) model, the mixture model, and the Eulerian model.The VOF ModelThe VOF model (described in Section 20.2) is a surface-tracking technique applied to a fixed Eulerian mesh. It is designed for two or more immiscible fluids where the position of the interface between the fluids is of interest. In the VOF model, a single set of momentum equations is shared by the fluids, and the volume fraction of each of the fluids in each computational cell is tracked throughout the domain. Applications of the VOF model include stratified flows , free-surface flows, filling, sloshing , the motion of large bubbles in a liquid, the motion of liquid after a dam break, the prediction of jet breakup (surface tension), and the steady or transient tracking of any liquid-gas interface.The Mixture ModelThe mixture model (described in Section 20.3) is designed for two or more phases (fluid or particulate). As in the Eulerian model, the phases are treated as interpenetrating continua. The mixture model solves for the mixture momentum equation and prescribes relative velocities to describe the dispersed phases. Applications of the mixture model include particle-laden flows with low loading, bubbly flows, sedimentation , and cyclone separators. The mixture model can also be used without relative velocities for the dispersed phases to model homogeneous multiphase flow.The Eulerian ModelThe Eulerian model (described in Section 20.4) is the most complex of the multiphase models in FLUENT. It solves a set of n momentum and continuity equations for each phase. Coupling is achieved through the pressure and interphase exchange coefficients. The manner in which this coupling is handled depends upon the type of phases involved; granular (fluid-solid) flows are handled differently than non-granular (fluid-fluid) flows. For granular flows , the properties are obtained from application of kinetic theory. Momentum exchange between the phases is also dependent upon the type of mixture being modeled. FLUENT's user-defined functions allow you tocustomize the calculation of the momentum exchange. Applications of the Eulerian multiphase model include bubble columns , risers , particle suspension, and fluidized beds .18.4 Choosing a Multiphase ModelThe first step in solving any multiphase problem is to determine which of the regimes described inSection 18.1 best represents your flow. Section 18.4.1 provides some broad guidelines for determining appropriate models for each regime, and Section 18.4.2 provides details about how to determine the degree of interphase coupling for flows involving bubbles, droplets, or particles, and the appropriate model for different amounts of coupling.18.4.1 General GuidelinesIn general, once you have determined the flow regime that best represents your multiphase system, you can select the appropriate model based on the following guidelines. Additional details and guidelines for selecting the appropriate model for flows involving bubbles, droplets, or particles can be found in Section 18.4.2.For bubbly, droplet, and particle-laden flows in which the dispersed-phase volume fractions are less than or equal to 10%, use the discrete phase model. See Chapter 19 for more information about the discrete phase model.For bubbly, droplet, and particle-laden flows in which the phases mix and/or dispersed-phase volume fractions exceed 10%, use either the mixture model (described in Section 20.3) or the Eulerian model (described in Section 20.4). See Sections 18.4.2 and 20.1 for details about how to determine which is more appropriate for your case.For slug flows, use the VOF model. See Section 20.2 for more information about the VOF model.For stratified/free-surface flows, use the VOF model. See Section 20.2 for more information about the VOF model.For pneumatic transport, use the mixture model for homogeneous flow (described in Section 20.3) or the Eulerian model for granular flow (described in Section 20.4). See Sections 18.4.2 and 20.1 for details about how to determine which is more appropriate for your case.For fluidized beds, use the Eulerian model for granular flow. See Section 20.4 for more information about the Eulerian model.For slurry flows and hydrotransport , use the mixture or Eulerian model (described, respectively, inSections 20.3 and 20.4). See Sections 18.4.2 and 20.1 for details about how to determine which is more appropriate for your case.For sedimentation, use the Eulerian model. See Section 20.4 for more information about the Eulerian model.For general, complex multiphase flows that involve multiple flow regimes, select the aspect of the flow that is of most interest, and choose the model that is most appropriate for that aspect of the flow. Note that the accuracy of results will not be as good as for flows that involve just one flow regime, since the model you use will be valid for only part of the flow you are modeling.18.4.2 Detailed GuidelinesFor stratified and slug flows, the choice of the VOF model, as indicated in Section 18.4.1, is straightforward. Choosing a model for the other types of flows is less straightforward. As a general guide, there are some parameters that help to identify the appropriate multiphase model for these other flows: the particulate loading, , and the Stokes number, St. (Note that the word ``particle'' is used in this discussion to refer to a particle, droplet, or bubble.)The Effect of Particulate LoadingParticulate loading has a major impact on phase interactions. The particulate loading is defined as the mass density ratio of the dispersed phase ( d) to that of the carrier phase ( c):The material density ratiois greater than 1000 for gas-solid flows, about 1 for liquid-solid flows, and less than 0.001 for gas-liquid flows. Using these parameters it is possible to estimate the average distance between the individual particles of the particulate phase. An estimate of this distance has been given by Crowe et al. [ 42]:where . Information about these parameters is important for determining how the dispersed phase shouldbe treated. For example, for a gas-particle flow with aparticulate loading of 1, the interparticle space is about 8; the particle can therefore be treated as isolated (i.e., very low particulate loading).Depending on the particulate loading, the degree of interaction between the phases can be divided into three categories:For very low loading, the coupling between the phases is one-way; i.e., the fluid carrier influences the particles via drag and turbulence, but the particles have no influence on the fluid carrier. The discrete phase, mixture, and Eulerian models can all handle this type of problem correctly. Since the Eulerian model is the most expensive, the discrete phase or mixture model is recommended.For intermediate loading, the coupling is two-way; i.e., the fluid carrier influences the particulate phase via drag and turbulence, but the particles in turn influence the carrier fluid via reduction in mean momentum and turbulence. The discrete phase, mixture, and Eulerian models are all applicable in this case, but you need to take into account other factors in order to decide which model is more appropriate. See below for information about using the Stokes number as a guide.For high loading, there is two-way coupling plus particle pressure and viscous stresses due to particles (four-way coupling). Only the Eulerian model will handle this type of problem correctly.The Significance of the Stokes NumberFor systems with intermediate particulate loading, estimating the value of the Stokes number can help you select the most appropriate model. The Stokes number can be defined as the relation between the particle response time and the system response time:where and t s is based on the characteristic length ( L s) and the characteristic velocity ( V s) of the system under investigation: .For , the particle will follow the flow closely and any of the three models (discrete phase, mixture, or Eulerian) is applicable; you can therefore choose the least expensive (the mixture model, in most cases), or themost appropriate considering other factors. For , the particles will move independently of the flowand either the discrete phase model or the Eulerian model is applicable. For , again any of the three models is applicable; you can choose the least expensive or the most appropriate considering other factors. ExamplesFor a coal classifier with a characteristic length of 1 m and a characteristic velocity of 10 m/s, the Stokes number is 0.04 for particles with a diameter of 30 microns, but 4.0 for particles with a diameter of 300 microns. Clearly the mixture model will not be applicable to the latter case.For the case of mineral processing, in a system with a characteristic length of 0.2 m and a characteristic velocity of 2 m/s, the Stokes number is 0.005 for particles with a diameter of 300 microns. In this case, you can choose between the mixture and Eulerian models. (The volume fractions are too high for the discrete phase model, as noted below.)Other ConsiderationsKeep in mind that the use of the discrete phase model is limited to low volume fractions. Also, the discrete phase model is the only multiphase model that allows you to specify the particle distribution or include combustion modeling in your simulation.。
Fluent软件模拟计算煤粉燃烧的机理及其模型实现的方式
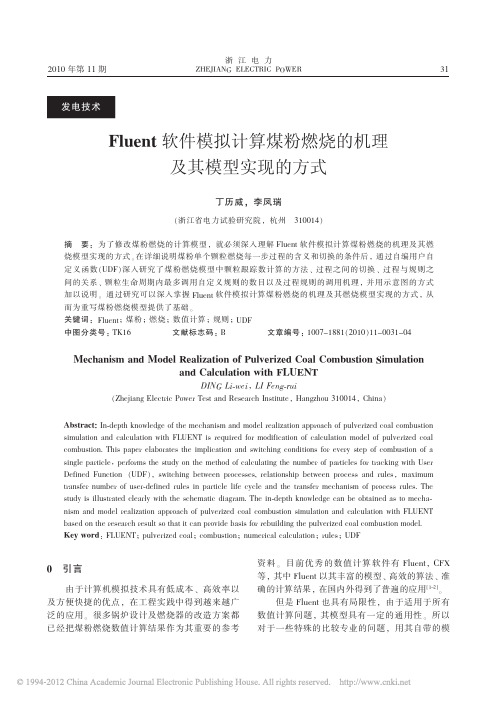
2010 年第 11 期
丁历威, 等: Fluent 软件模拟计算煤粉燃烧的机理及其模型实现的方式
33
跟踪颗粒数=1×(Number of Tries) (1) 2.2 Group 类型
Group 类型是指煤粉颗粒按照直径分成多股 煤粉流, 每股煤粉流之间直径、质量、 流量、 位置 都各不同, 然后每股煤粉流按照 1 个 Single 类型 来处理, 所以该类型下 Fluent 跟踪的颗粒数为: 跟踪颗粒数=(Number of Group)×(Number of Tries)
(2) 2.3 Surface 类型
Surface 类型是指煤粉颗粒根据入口面网格分 成多股煤粉流。 每 1 个面网格相当于 1 个 Group, 所以该类型 Fluent 跟踪的颗粒数为:
跟踪颗粒数=(Number of Face)×(Number of Group)×(Number of Tries) (3)
率很慢, 扩散过来的氧量远远超过反应所需的氧 量, 这时燃烧速率与反应有关。
(2)当温度超过 1 400℃时, 由于化学反应速 率常数随着温度升高急剧增大, 致使反应所消耗 的氧量远远超过扩散过来的氧量, 这时燃烧速率 主要由氧气的扩散速度决定。
(3)当温度在 1 000~1 400℃之间 , 燃烧速率 受到反应速率和氧气扩散速率双重影响。 1.4 灰分的吸热或者冷却(Fourth Law)
烧模型实现的方式。在详细说明煤粉单个颗粒燃烧每一步过程的含义和切换的条件后, 通过自编用户自
定义函数(UDF)深入研究了煤粉燃烧模型中颗粒跟踪数计算的方法、 过程之间的切换、 过程与规 则之
间的关系、 颗粒生命周期内最多调用自定义规则的数目以及过程规则的调用机理, 并用示意图的方式
FLUENT 6.3 使用手册
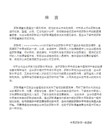
Fluent 6.3Fluent 6.3Fluent 6.3Fluent 6.3使用手册使用手册使用手册使用手册 第第第第9999页页页页((((共共共共20202020页页页页)))) 这一部分表示包含悬挂节点的网格面的层级。其格式如下: 其中 face-id0:该部分中第一个父面的指针 face-id1:该部分中最后一个父面的指针 parent-zone-id:包含父面区域的ID child-zone-id:包含子面区域的ID number-of-kids:父面包含的子面个数 kid-id-n:子面的ID 以上这些都是十六进制格式。 B.3.9单元结构 这一部分表示包含悬挂节点的网格的单元的层级。其格式如下: 其中 cell-id0:该部分中第一个父单元的指针 cell-id1:该部分中最后一个父单元的指针 parent-zone-id:包含父单元区域的ID child-zone-id:包含子单元区域的ID number-of-kids:父单元包含的子单元个数 kid-id-n:子单元的ID 以上这些都是十六进制格式。
带你发现Fluent电池模型仿真计算
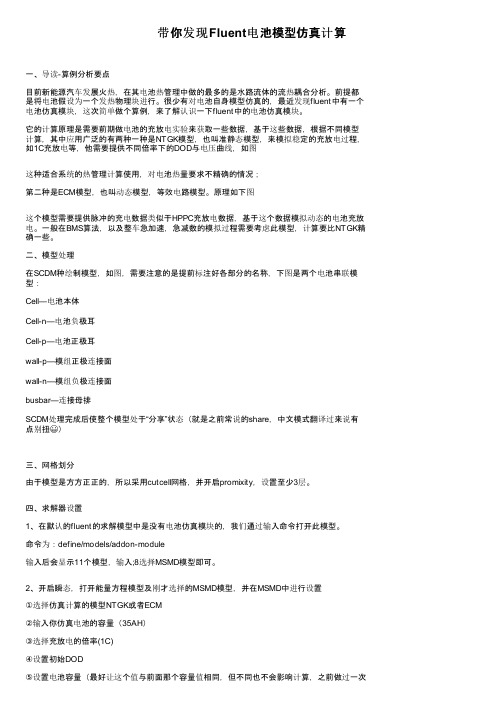
带你发现Fluent电池模型仿真计算一、导读-算例分析要点目前新能源汽车发展火热,在其电池热管理中做的最多的是水路流体的流热耦合分析。
前提都是将电池假设为一个发热物理块进行。
很少有对电池自身模型仿真的,最近发现fluent中有一个电池仿真模块,这次简单做个算例,来了解认识一下fluent中的电池仿真模块。
它的计算原理是需要前期做电池的充放电实验来获取一些数据,基于这些数据,根据不同模型计算,其中应用广泛的有两种一种是NTGK模型,也叫准静态模型,来模拟稳定的充放电过程,如1C充放电等,他需要提供不同倍率下的DOD与电压曲线,如图这种适合系统的热管理计算使用,对电池热量要求不精确的情况;第二种是ECM模型,也叫动态模型,等效电路模型。
原理如下图这个模型需要提供脉冲的充电数据类似于HPPC充放电数据,基于这个数据模拟动态的电池充放电。
一般在BMS算法,以及整车急加速,急减数的模拟过程需要考虑此模型,计算要比NTGK精确一些。
二、模型处理在SCDM种绘制模型,如图,需要注意的是提前标注好各部分的名称,下图是两个电池串联模型:Cell—电池本体Cell-n—电池负极耳Cell-p—电池正极耳wall-p—模组正极连接面wall-n—模组负极连接面busbar—连接母排SCDM处理完成后使整个模型处于“分享”状态(就是之前常说的share,中文模式翻译过来说有点别扭 )三、网格划分由于模型是方方正正的,所以采用cutcell网格,并开启promixity,设置至少3层。
四、求解器设置1、在默认的fluent的求解模型中是没有电池仿真模块的,我们通过输入命令打开此模型。
命令为:define/models/addon-module输入后会显示11个模型,输入;8选择MSMD模型即可。
2、开启瞬态,打开能量方程模型及刚才选择的MSMD模型,并在MSMD中进行设置①选择仿真计算的模型NTGK或者ECM②输入你仿真电池的容量(35AH)③选择充放电的倍率(1C)④设置初始DOD⑤设置电池容量(最好让这个值与前面那个容量值相同,但不同也不会影响计算,之前做过一次对比,他们具体有是什么差异目前没有搞明白,如果有明白可以留言讨论,不胜感激!)⑥根据NTGK与ECM所提供的不同实验数据,计算所得的值,具体计算方法可参考帮助文件有详细介绍。
coal fluent能量燃烧计算
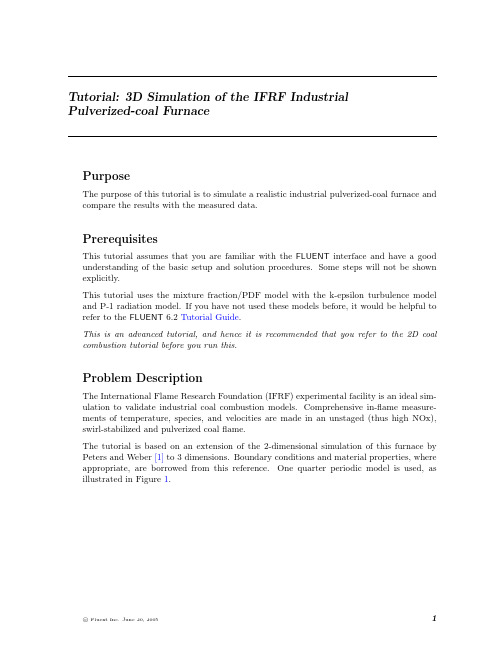
Tutorial: 3D Simulation of the IFRF Industrial Pulverized-coal FurnacePurposeThe purpose of this tutorial is to simulate a realistic industrial pulverized-coal furnace and compare the results with the measured data.PrerequisitesThis tutorial assumes that you are familiar with the FLUENT interface and have a good understanding of the basic setup and solution procedures. Some steps will not be shown explicitly. This tutorial uses the mixture fraction/PDF model with the k-epsilon turbulence model and P-1 radiation model. If you have not used these models before, it would be helpful to refer to the FLUENT 6.2 Tutorial Guide. This is an advanced tutorial, and hence it is recommended that you refer to the 2D coal combustion tutorial before you run this.Problem DescriptionThe International Flame Research Foundation (IFRF) experimental facility is an ideal simulation to validate industrial coal combustion models. Comprehensive in-ame measurements of temperature, species, and velocities are made in an unstaged (thus high NOx), swirl-stabilized and pulverized coal ame. The tutorial is based on an extension of the 2-dimensional simulation of this furnace by Peters and Weber [1] to 3 dimensions. Boundary conditions and material properties, where appropriate, are borrowed from this reference. One quarter periodic model is used, as illustrated in Figure 1.c Fluent Inc. June 20, 200513D Simulation of the IFRF Industrial Pulverized-coal FurnaceFigure 1: Problem FigurePreparation1. Copy the les, ifrf.msh and ifrf.c.c to your working directory. 2. Start the 3D version of FLUENT.Setup and SolutionBefore starting to set up the problem, you have to decide which combustion model to use. Here, choose the equilibrium mixture fraction model, as opposed to the Eddy-Dissipation model. The principal assumptions for this case are chemical equilibrium and modeling the devolatilization and char o-gases as a single mixture. The coal used is Saar Gottelborn hvBb. The proximate analysis is (weight %, dry) 55% volatiles, 36.7% xed carbon, and 8.3% ash. The ultimate analysis is (weight %, dry-ashfree (daf )) 80.36% C, 5.08% H, 12.17% O, 1.45% N, and 0.94% S. The LCV is (daf ) 32.32 MJ/kg.2c Fluent Inc. June 20, 20053D Simulation of the IFRF Industrial Pulverized-coal FurnaceStep 1: Grid 1. Read the mesh le (ifrf.msh). 2. Check and scale the grid. 3. Display the grid.Y X ZGridFLUENT 6.2 (3d, segregated, lam)Figure 2: GridStep 2: Models 1. Retain the default solver settings. 2. Enable the standard k-epsilon (2 eqn) turbulence model. 3. Select Non-Premixed Combustion as the species model. (a) Under PDF Options, enable Create Table. The original Species Model panel expands with options for PDF table inputs. (b) Click the Chemistry tab and enable Non-Adiabatic. (c) Under Options, enable Empirical Fuel Stream, and specify the Empirical Parameters as follows: Empirical Parameters Fuel Lower Caloric Value Fuel Specic Heat Values 3.232e+07 1100c Fluent Inc. June 20, 200533D Simulation of the IFRF Industrial Pulverized-coal Furnace(d) Dene the boundary species (species entering the domain as part of the fuel or oxidizer composition). i. Click the Boundary tab. Under Species Units, select Mass Fraction. ii. Dene the composition of fuel as follows: Species c h o n Species Fraction 0.8036 0.0508 0.1217 0.0239For simplifying modeling, the sulfur content of the coal is combined into the nitrogen mass fraction. iii. Dene the composition of oxidizer as follows: Species o2 n2 Species Fraction 0.2329 0.7671iv. Specify the temperature for Fuel and Oxidizer as 373 and 573 respectively. (e) Click the Table tab and retain the default values for the Table Parameters. (f) Calculate the PDF table. 4. Select P1 as the radiation model. To choose an appropriate radiation model, calculate the optical thickness, which is the mean beam length (about 2m) multiplied by the absorption coecient (around 1 per m3 for hydrocarbon combustion). Since this optical thickness is greater than unity, the P1 model is appropriate. 5. Dene the discrete phase model. (a) Set the Max. Number of Steps to 25000. This is the maximum number of steps that each particle will track. This number is not known before hand. Increase the number of steps if too many tracks are incomplete, or decrease if particles are trapped in a re-circulation zone and computational times are excessive. The error induced by the incomplete tracks will be negligible because coal particle devolatilization and subsequent char combustion occur relatively close to the burner, and ash interaction with the gas-phase is relatively not important and far from the burning zone. (b) Set the Step Length Factor to 20. This option sets the particle step length to roughly the cell characteristic length (cube root of the cell volume) divided by the Step Length Factor. Specify the step length directly since cells are usually clustered in the near-burner zone where the gradients are steepest and resolution is needed. (c) Retain the default values for the other parameters. 4c Fluent Inc. June 20, 20053D Simulation of the IFRF Industrial Pulverized-coal FurnaceStep 3: Materials 1. Modify the mixture properties. (a) Select wsggm-cell-based for Absorption Coecient and set the Scattering Coecient to 0.15. Retain the default values for the other parameters. Step 4: Operating Conditions 1. Retain the default operating conditions. Step 5: Compile the Interpreted UDFs In a separate editor, view the ifrf.c.c UDF and familiarize yourself with its contents. When you understand the structure and function of the UDF, interpret the UDF. For more information on interpreted UDF's, refer to Section 7.2: Interpreted UDFs, in the FLUENT UDF Manual. 1. Compile the UDF using the Interpreted UDFs panel. Dene → User-Dened → Functions →Interpreted... (a) Enter the name of the C function (ifrf.c.c) under Source File Name. (b) Specify the C preprocessor to be used in the CPP Command Name eld. (c) Keep the default Stack Size setting of 10000, unless the number of local variables in your function will cause the stack to overow. In this case, set the Stack Size to a number that is greater than the number of local variables used. (d) Select the Use Contributed CPP option if you want to use the C preprocessor that Fluent Inc. has supplied, instead of using your own. (e) Click Interpret. (f) When the interpretation is over, close the panel. If you keep the panel open, the Compile button can be used repeatedly while you proceed with debugging the function and continue to compile until no errors are reported.c Fluent Inc. June 20, 200553D Simulation of the IFRF Industrial Pulverized-coal FurnaceStep 6: Boundary Conditions The CFD solution for reacting ows can be sensitive to the boundary conditions, in particular, the incoming velocity eld and the heat transfer through the walls. Here, use the UDF proles to specify the velocity inlet v-2, and the wall temperature for the walls w-5, w-6, and w-7. The latter approach of xing the wall temperature to measurements is common in furnace simulation. It is used to avoid modeling the wall convective and radiative heat transfer. Linear and parabolic polynomial ts are used. 1. Set the boundary conditions for the v-1 zone. The transport air mass ow rate is 421 kg/h at a temperature of 343 K and at atmospheric pressure, the density is 1.03kg/m3 . Since the inlet area is 0.001228m2 (we are modeling one-fourth of the geometry here), the inlet velocity is 23.11 m/s. (a) Set the Velocity Magnitude and Temperature to 23.11 and 343 K respectively. (b) Select Intensity and Hydraulic Diameter as the Turbulence Specication Method and set the Hydraulic Diameter to 0.013. (c) Retain the default values for the other parameters. 2. Set the boundary conditions for the v-2 zone. (a) Select Components as the Velocity Specication Method and Cylindrical (Radial, Tangential, Axial) as the Coordinate System. (b) Select udf vinlet2wvel for Tangential-Velocity and udf vinlet2uvel for Axial-Velocity. Set the Temperature to 573. The axial and swirl velocity proles are determined by the UDF functions udf vinlet2uvel and udf vinlet2wvel. (c) Select Intensity and Hydraulic Diameter as the Turbulence Specication Method and set the Hydraulic Diameter to 0.047. (d) Retain the default values for the other parameters. 3. Set the boundary conditions for the p-1 zone. (a) Set the Backow Total Temperature to 1200. (b) Select Intensity and Hydraulic Diameter as the Turbulence Specication Method and set the Backow Hydraulic Diameter to 0.84. Note: These values will be used only if there is backow. Though we do not expect a backow in the nal solution, backow may occur during iteration to the nal solution. Hence, it is a good practice to set these values. (c) Retain the default values for the other parameters.6c Fluent Inc. June 20, 20053D Simulation of the IFRF Industrial Pulverized-coal Furnace4. Set the boundary conditions for the wall zones as shown in the following table: Zone Name w-1 w-2 w-3 w-4 w-5 w-6 w-7 w-8 w-9 Temperature 343 573 873 1273 udf wall5temp udf wall6temp udf wall7temp 1323 1073 Internal Emissivity 0.6 0.6 0.6 0.6 0.6 0.6 0.6 0.6 0.65. Set the Periodic Type for the periodic zone to Rotational. Step 7: Injections Dene the Discrete Phase (coal particle) injection properties. Although this is not required for the rst non-reacting solution stage, it is better to complete the setup. 1. Set the Injection Type to surface and select v-1 for Release From Surfaces. 2. Set the Particle Type to Combusting and select rosin-rammler for Diameter Distribution. By default, anthracite will be selected as the combusting particle. At a latter stage, change this to gottelborn-hv. 3. Under Turbulent Dispersion, enable the Stochastic Model and set the Number of Tries equal to 3. Stochastic tracking is used to model turbulent dispersion of coal particles. It is critical for realistic modeling. We have chosen an arbitrary number of 3 stochastic tracks, which is a balance between accuracy (increased number of tracks), and computational speed (reduced number of tracks). If the solution diverges after particle tracking, you might consider increasing the number of tracks (and/or reducing the DPM underrelaxation factor), to enhance stability.c Fluent Inc. June 20, 200573D Simulation of the IFRF Industrial Pulverized-coal Furnace4. Under Point Properties, set the parameters as shown in the following table: Parameter Z-Velocity Temperature Total Flow Rate Min. Diameter Max. Diameter Mean Diameter Spread Parameter Number of Diameters Value 23.11 343 0.01826 1e-06 0.003 4.5e-05 1.36 65. Modify the properties of the combusting particle. Dene →Materials... (a) Select combusting-particle in the Material Type scroll down list. (b) Change the name of the combusting particle from anthracite to gottelborn-hv. (c) Set the values for the properties as follows: Parameter Density Cp Latent Heat Vaporization Temperature Volatile Component Fraction Binary Diusivity Combustible Fraction Value 1000 1100 0 300 55.02 3e-05 36.7(d) In the same list, select kinetics/diffusion-limited in the Combustion Model drop-down list and set the following parameters: Parameter Kinetics-Limited Rate Pre-Exponential Factor Kinetics-Limited Rate Activation Energy Value 6.7 1.1382e+08Coal burning will be initiated immediately by setting the coal Vaporization Temperature to 300 K (which is less than the initial coal temperature), and the Devolatilization Model to a constant. This is not an exclusive approach to 'lighting the re'. Other procedures such as patching in a hot temperature zone or starting from a combusting gas phase solution might be more ecient. When a stable ame is established, change the properties back to their appropriate values. For steady-state simulations, the nal converged solution is independent of the solution path and initial conditions.8c Fluent Inc. June 20, 20053D Simulation of the IFRF Industrial Pulverized-coal FurnaceStep 8: Solution (Non-reacting Flow) It is recommended to rst obtain a non-reacting ow solution for coal combustion. Despite the large density variations that occur in reacting ows, the velocity and turbulence elds are similar for reacting and isothermal combustors. The trajectory of the DPM coal particles is critical and is sensitive to the velocity and turbulence elds. Hence, to achieve convergence, it is necessary to start from a non-reacting solution. 1. Disable Energy, P1, and Pdf equations and set the pressure discretization to PRESTO!. This is often useful for buoyant ows where velocity vectors near walls may not align with the wall due to the assumption of uniform pressure in the boundary layer for these highly buoyant cases. For the combustor under study, the buoyancy forces are negligible, and the dierence between PRESTO! and standard dierencing is minimal. 2. Initialize the solution and compute from all-zones. 3. Set the initial value for temperature to 2000. 4. Enable the plotting of residuals during calculation. 5. Start by requesting 99 iterations. Save the data le. It is not necessary to solve for a completely converged non-reacting solution. You just want a velocity and turbulence eld to send the coal particles in the correct directions.Residuals continuity x-velocity y-velocity z-velocity k epsilon1e+011e+001e-011e-021e-031e-04Y X Z0102030405060708090100IterationsScaled ResidualsFLUENT 6.2 (3d, segregated, pdf20, ske)Figure 3: Scaled ResidualsWith the non-reacting solution, you are ready to release the coal particles. 6. For the discrete phase model, enable Interaction with Continuous Phase and set the Number of Continuous Phase Iterations per DPM Iteration to 20. 9c Fluent Inc. June 20, 20053D Simulation of the IFRF Industrial Pulverized-coal Furnace7. Enable the Energy, P1 and Pdf equations. Set the under-relaxation factors for the following parameters: Parameter Pressure Momentum P1 Discrete Phase Sources Value 0.5 0.5 0.975 0.25With sudden heat release, the velocity and turbulence elds may change very quickly and cause divergence. To control this, decrease the Momentum under-relaxation factor. The default value of 0.8 for P1 is conservative and will require a large number of iterations for convergence. This case will diverge when the P1 under-relaxation factor is set to 1, so a value of 0.975 is determined by trial and error. The Discrete Phase Sources under-relaxation factor is set to 0.25, which is more stable than the default of 0.5. 8. Request for an additional 20 iterations and then save the data le. This corresponds to one DPM iteration with our automatic ignition coal.Residuals continuity x-velocity y-velocity z-velocity energy k epsilon fmean fvar p11e+011e+001e-011e-021e-031e-041e-05Y X Z020406080100120IterationsScaled ResidualsFLUENT 6.2 (3d, segregated, pdf20, ske)Figure 4: Scaled Residuals9. Modify the properties of the combusting particle. Set the values for the following parameters: (a) Set Vaporization Temperature to 773 K.10c Fluent Inc. June 20, 20053D Simulation of the IFRF Industrial Pulverized-coal Furnace(b) Select single-rate under Devolatilization Model drop-down list and set the following parameters: Parameter Pre-Exponential Factor Activation Energy Value 2e+05 7.4e+0710. Request for an additional 200 iterations. Save the data le. It is a good practice to start with rst order spatial discretization. It is important to try and obtain a second (or higher) order solution. The solver will generally converge unless the grid has excessive skew, or the equations are numerically sti. 11. For the discrete phase model, set the Number of Continuous Phase Iterations per DPM Iteration to 40. 12. Set the under-relaxation factors for the following parameters: Parameter Momentum Density Turbulent Viscosity Value 0.2 0.8 0.713. Set the discretization for Momentum, Turbulence Kinetic Energy, Turbulence Dissipation Rate, Energy, Mean Mixture Fraction, and Mixture Fraction Variance to Second Order Upwind. 14. Request for an additional 500 iterations. Save the data le.c Fluent Inc. June 20, 2005113D Simulation of the IFRF Industrial Pulverized-coal FurnaceResiduals continuity x-velocity y-velocity z-velocity energy k epsilon fmean fvar p11e+01 1e+00 1e-01 1e-02 1e-03 1e-04 1e-05 1e-06 1e-07Y Z X0100200300400500600700800IterationsScaled ResidualsFLUENT 6.2 (3d, segregated, pdf20, ske)Figure 5: Scaled Residuals15. Dene the NOx Model. Note: The nal task is to model the NOx eld. Since NOx is a trace species, it does not aect the ow and can also be solved in the postprocessing step. Dene → Models → Species →NOx... (a) Enable the models Thermal NO and Fuel NO. (b) Under Turbulence Interaction, select Mixture Fraction for PDF Mode and set the Beta PDF Points to 25. (c) Click the Fuel tab, select Solid for Fuel Type, and set Volatile N Mass Fraction, Char N Mass Fraction, and BET Surface Area to 0.0188, 0.00805, and 25000 respectively. 16. For the discrete phase model, set the Number of Continuous Phase Iterations per DPM Iteration to 0. 17. Disable all the equations except NO and HCN. Set the under-relaxation factors for NO and HCN to 1 and select Second Order Upwind for their discretization schemes. 18. Set the Convergence Criterion for NO and HCN to 1e-06. 19. Request for 20 iterations and then save the data le.12c Fluent Inc. June 20, 20053D Simulation of the IFRF Industrial Pulverized-coal FurnaceResiduals pollut_no pollut_hcn1e-011e-021e-031e-041e-051e-061e-071e-08Y Z X760762764766768770772774776778780IterationsScaled ResidualsFLUENT 6.2 (3d, segregated, pdf20, ske)Figure 6: Scaled ResidualsStep 9: Postprocessing For both cases, all the residuals should be below Fluent's convergence criteria, which is 1e-3 for all variables, except Energy and P1. These equations are considered converged when the residual is below 1e-6. Despite the indication of convergence, it is a good practice to check the net in ux and out ux balance. 1. Compute the gas phase mass uxes through all the boundaries. Report →Fluxes... (a) Select all the zones under Boundaries and click Compute. 2. Calculate the net mass transfer to the gas phase from the discrete phase coal particles. Report →Volume Integrals... (a) Under Report Type, enable Sum, and under Cell Zones, select uid. (b) Select Discrete Phase Model... and DPM Mass Source from the Field Variable drop-down lists and click Compute. 3. Compute the gas phase energy uxes through all the boundaries. Report →Fluxes... (a) Under Options, enable Total Heat Transfer Rate. (b) Select all the zones under Boundaries and click Compute.c Fluent Inc. June 20, 2005133D Simulation of the IFRF Industrial Pulverized-coal Furnace4. Calculate the net mass transfer to the gas phase from the discrete phase coal particles. Report →Volume Integrals... (a) Under Report Type, enable Sum, and under Cell Zones, select uid. (b) Select Discrete Phase Model... and DPM Enthalpy Source from the Field Variable drop-down lists and click Compute. The sum of the net gas phase and discrete phase heat transfer should be much smaller than a representative heat ux, such as the heat ux out of the outlet boundary. Also, this energy balance should improve with additional iterations. 5. Display contours of Static Temperature (Figure 7). 6. Display contours of Velocity Magnitude (Figure 8). 7. Display contours of Mass Fraction of co2 (Figure 9), o2 (Figure 10), and NO (Figure 11) on x=0m plane.2250 2154 2059 1964 1868 1773 1678 1582 1487 1392 1296 1201 1106 1010 915 820 724 629 534 438 343XY ZContours of Static Temperature (k)FLUENT 6.2 (3d, segregated, pdf20, ske)Figure 7: Contours of Static Temperature14c Fluent Inc. June 20, 20053D Simulation of the IFRF Industrial Pulverized-coal Furnace73 69 66 62 58 55 51 47 44 40 36 33 29 26 22 18 15 11 7 4 0XY ZContours of Velocity Magnitude (m/s)FLUENT 6.2 (3d, segregated, pdf20, ske)Figure 8: Contours of Velocity Magnitude2.329e-01 2.212e-01 2.096e-01 1.979e-01 1.863e-01 1.746e-01 1.630e-01 1.514e-01 1.397e-01 1.281e-01 1.164e-01 1.048e-01 9.314e-02 8.150e-02 6.986e-02 5.821e-02 4.657e-02 3.493e-02 2.329e-02 Y Z 1.164e-02 X 0.000e+00Contours of Mass fraction of co2FLUENT 6.2 (3d, segregated, pdf20, ske)Figure 9: Contours of Mass Fraction of co2c Fluent Inc. June 20, 2005153D Simulation of the IFRF Industrial Pulverized-coal Furnace2.329e-01 2.213e-01 2.096e-01 1.980e-01 1.863e-01 1.747e-01 1.630e-01 1.514e-01 1.397e-01 1.281e-01 1.165e-01 1.048e-01 9.316e-02 8.152e-02 6.987e-02 5.823e-02 4.658e-02 3.494e-02 2.329e-02 Y Z 1.165e-02 X 0.000e+00Contours of Mass fraction of o2FLUENT 6.2 (3d, segregated, pdf20, ske)Figure 10: Contours of Mass Fraction of o24.074e-03 3.870e-03 3.667e-03 3.463e-03 3.259e-03 3.056e-03 2.852e-03 2.648e-03 2.444e-03 2.241e-03 2.037e-03 1.833e-03 1.630e-03 1.426e-03 1.222e-03 1.019e-03 8.148e-04 6.111e-04 4.074e-04 Y Z 2.037e-04 X 0.000e+00Contours of Mass fraction of NOFLUENT 6.2 (3d, segregated, pdf20, ske)Figure 11: Contours of Mass Fraction of NO16c Fluent Inc. June 20, 20053D Simulation of the IFRF Industrial Pulverized-coal FurnaceResultsTime averaged ow eld values were collected at various locations in the combustor. In addition, plot lines at z=0.25m and z=0.85m are incorporated in the grid. You can generate radial proles and axial plots at 0.25 m and 0.85 m (see Figure 1). The simulation results can be compared with the available experimental data (temperature, velocity, species etc.). The experimental data is available in the reference given below.References[1] Peters, A.F. and Weber, R. (1997), Mathematical Modeling of a 2.4 MW Swirling, Pulverized Coal Flame, Combustion Science and Technology, 122, 131.c Fluent Inc. June 20, 200517。
FLUENT6(可编辑)
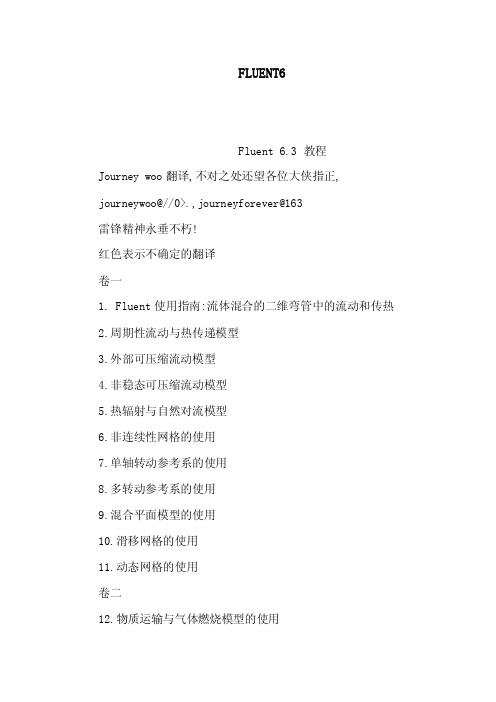
FLUENT6Fluent 6.3 教程Journey woo翻译,不对之处还望各位大侠指正, journeywoo@//0>.,journeyforever@163雷锋精神永垂不朽!红色表示不确定的翻译卷一1. Fluent使用指南:流体混合的二维弯管中的流动和传热2.周期性流动与热传递模型3.外部可压缩流动模型4.非稳态可压缩流动模型5.热辐射与自然对流模型6.非连续性网格的使用7.单轴转动参考系的使用8.多转动参考系的使用9.混合平面模型的使用10.滑移网格的使用11.动态网格的使用卷二12.物质运输与气体燃烧模型的使用13.非预先混合燃烧模型的使用14.表面化学反应模型15.雾状液体蒸发模型16.VOFvolume of fluid流体体积函数模型的使用17.气蚀模型18.混合与欧拉多元模型19.欧拉多元模型在颗粒流中的使用20.凝固过程模拟21.有传热欧拉多元模型在颗粒流中的使用22.后处理23.汽轮机后处理24.并行处理目录1. Fluent使用指南:有流体混合的二维弯管中的流动和传热介绍前言问题描述求解过程准备第一步:网格第二部:模型第三步:材料第四步:边界条件第五步:求解第六步:展示初步解决方案第七部:启用二阶离散第八步:调整网格总结2.周期性流动与热传递模型介绍前言问题描述求解过程准备第一步:网格第二部:模型第三步:材料第四步:边界条件第五步:求解第六步:后处理总结进一步改善3.外部可压缩流动模型如何使用这本手册这本手册里有什么?这本Fluent教学指导包括了一些教你如何使用Fluent来解决各种问题的教程.在每一个教程中,特征与问题的设置和后处理相关可以被证实.教程1是一个十分细化的教程,专门为Fluent的入门者而设置的.这个教程在问题的设置、求解、后处理等各个步骤上讲得十分的详细.剩下的教程是为那些已经阅读过了教程1或是对Fluent和其接口比较熟悉的读者设置的.在那些教程中,有些步骤没有详细讲解.所有的教程中都包括后处理的指导,但是教程23讲的完全是标准的后处理过程,教程24是专门讲汽轮机问题的后处理.这本手册中使用到的文件哪里去找?这本手册中的每个教程都有现成的网格文件(也就是说,教程的网格生成那一步提供了网格文件).你能在FluentCD中找到对应的网格文件(和与这个教程相关的文件).每一个教程的“准备”环节会告诉你在哪里找到需要的文件.(注意,教程23、24、25要用现成的算例与数据文件)有一些比较复杂的教程可能需要大量的计算时间,如果你不想等计算结束而想马上看到计算的结果,你可以直接使用算例和数据文件case?data files,这个文件在Fluent CD中,就在你找到mesh文件的文件夹里.mesh文件和fluent帮助下载地////.这本手册怎么使用?根据你对CFD(计算流体力学)和Fluent软件的熟悉程度,你可以有很多方法来使用这本手册.初学者如果你是个Fluent的初学者,你应当先看看教程1,熟悉Fluent的接口、基本设置和求解的步骤.当你要处理的问题与教程有共同点,你也可以参考教程.比如说,你解决的问题用到了非预混燃烧模型,你就可以参考教程14.就算这个教程和你要解决的问题没关系,你也可以参照教程来熟悉Fluent各种功能的使用,比如用户字段功能、网格尺度等等.如果要学习关于后处理的方法,你可以参照教程23,教程23是完全关于后处理的(虽然其他的教程也包括后处理过程).关于与汽轮机有关的问题的后处理,请参照教程24.有经验者如果你对Fluent比较熟悉了,当你要处理的问题与教程有共同点,你也可以参考教程.比如说,你解决的问题用到了非预混燃烧模型,你就可以参考教程14.就算这个教程和你要解决的问题没关系,你也可以参照教程来熟悉Fluent各种功能的使用,比如用户字段功能、网格尺度等等.如果要学习关于后处理的方法,你可以参照教程23,教程23是完全关于后处理的(虽然其他的教程也包括后处理过程).关于与汽轮机有关的问题的后处理,请参照教程24.这本手册中的图标这本手册使用了一些图标来帮助您的学习.信息图标标记着一些重要的信息警告图标标记着警号警告信息不同字体表明用户界面菜单项和文本界面菜单项比如当要只是当前出现在屏幕的是什么或者你需要在指定的窗口或面板输入数据的时候也会出现不同的字体.每个教程中每个步骤的演示用的都是规范的字体,而每个步骤的附加信息是用斜体字表示的.字符边框表示的是你要打开的命令或者面板是属于哪个菜单选项.比如:Define Boundary Conditions表示Boundary Conditions菜单可以在Define选项的下拉菜单中找到.教程一:Fluent入门:有流体混合的二维弯管中的流动和传热介绍:这个教程演示了一个三维湍流的弯管中流体混合过程的流体流动和传热问题的相关设置和求解过程.这种过程在发电厂或者管道系统中可以见到.为了正确的设计接头部分,混合部位的流场与温度场的预估是十分重要的.本教程演示了如何执行以下操作:如何使用Fluent读取现成的网格文件使用混合部位确定的几何形状和流体性质强迫对流的湍流问题如何设定物性参数和边界条件输出残差示意图的计算初始化使用压力基求解器来求解使用Fluent的图形输出工具来直观上查看流场与温度场使用二阶离散器来更好的计算温度场在温度梯度的基础上调整网格以更好的计算温度场前提条件这个教程是假定您完全没有接触过Fluent,其中每一个步骤都将有十分详细的介绍.问题描述要解决的问题如图1.1所示.20℃的冷流体从直径大的一端流入管道中,40℃的温热流体从直径小的位于弯头处的一端流入管中,二者混合.管道的尺寸是用英尺来标注的,而流体的参数和边界条件是用国际单位制表示的.大口径的入口处雷诺数为50800,因此我们要建立一个湍流模型.开始与求解准备1.从Fluent用户中心//.下载introduction.zip到您的工作文件夹中,这个文件可以在Fluent产品页的文件连接中找到.或者您可以从Fluent文件CD中将introduction.zip拷贝到您的工作文件夹中.对于Linux/UNIX系统,你可以将CD插入CD-ROM中,然后按如下路径进入文件夹:/cdrom/fluent6.3/help/tutfiles/上面路径中的cdrom换成您的CD-ROM的名称,比如说E对于Linux/UNIX系统,你可以将CD插入CD-ROM中,然后按如下路径进入文件夹:cdrom:\fluent6.3\help\tutfiles\上面路径中的cdrom换成您的CD-ROM的名称,比如说E2.解压缩introduction.zip文件elbow.msh文件可以在解压缩后产生的introduction文件夹中找到.3.打开FLUENT选择3D3d视图第一步:网格1.读取网格文件elbow.mesh.File-Read-case…a在解压缩产生的introduction文件夹中单击选择elbow.mesh文件.b单击OK读取文件关闭文件选择对话框.Note:当FLUENT读取了网格文件之后,控制面板上将会显示转换进程的信息.FLUENT会报告已经读取了13852个六面体计算单元和一些有着不同zone identifiers边界面.2.检查网格Grid-Check…Grid CheckGrid CheckDomain Extents:x-coordinate: min m -8.000000e+000, m 8.000000e+000 y-coordinate: min m -9.134633e+000, m 8.000000e+000 z-coordinate: min m 0.000000e+000, m 2.000000e+000Volume statistics:minimum volume m3: 5.098261e-004 imum volume m3: 2.330738e-002total volume m3: 1.607154e+002 Face area statistics:minimum face area m2: 4.865882e-003 imum face area m2: 1.017924e-001 Checking number of nodes per cell. Checking number of faces per cell. Checking thread pointers.Checking number of cells per face. Checking face cells.Checking bridge faces.Checking right-handed cells. Checking face handedness.Checking face node order.Checking element type consistency. Checking boundary types:Checking face pairs.Checking periodic boundaries. Checking node count.Checking nosolve cell count.Checking nosolve face count.Checking face children.Checking cell children.Checking storage.Done.Note: The minimum andNote:在不同的平台上运行时,最大值imum和最小值minimum可能有些不同.网格检查的结果以国际单位制中的米来表示最大的和最小的x与y的值,以及一些其他的网格特征检查的结果.如果网格出错,将会在此时给出错误的信息errors.特别要说明的是,网格的最小体积一定要大于零,否则fluent不能开始计算.因为本例中图形采用英尺作为单位,下一步,您需要改变网格的比例尺来使用英尺作为单位.3.网格比例尺Grid-Scale…a在单位转换Unit Conversion的窗口中网格创建于Grid Was Created In选项的下拉菜单中选择英尺这一项.先点击向下的箭头按钮,然后点击in选项.b单击Scale按钮来转换网格的单位.Scale按钮只要点击一次就可以了区域宽度的值是以国际单位制中的米作为单位的.c点击change length unit按钮,将英尺作为本计算中的长度单位.d确定您的区域尺寸像上图的面板中显示的一样.e点击Close按钮关闭单位转换面板.现在网格的设置正确了,而且计算过程中使用的长度单位也变成了英尺.Note:因为本例中除了长度以外使用的都是国际单位制,所以本问题中其他的方面就不需要更改单位了.通过刚才的操作,您已经将长度单位改成了英尺,如果您想用其他的长度单位,比如说milimetres,您需要在Define选项的下拉菜单中打开set unit面板,进行相应的修改.4.显示网格图1.2Display-Grid…a保留Surfaces选项单里的缺省选项,除了default-interior 这一项.Note:如果一个选项被选择了那么它会用高光表示,如果没有选择就没有高光,你可以单击来选择这些选项.b单击Display,打开图形显示窗口显示网格.c关闭Grid Display面板.另外:在图形显示窗口中,你可以用右键点击网格的某处来获取网格的信息,网格的信息会在Fluent控制窗口中显示出来,包括这个区域的名字.这个特点在当你有很多有相同类型的区域的时候十分有用,如果你想要快速的区分这些区域,你可以使用这个功能.转动视角拖动鼠标左键即可转动视角,当视角符合您的要求的时候,松开鼠标即可.改变视野用鼠标中键在任意处单击一下,图形会以此处为中点来显示.放大某部分显示按住鼠标的中键,向右拖动,向上向下都可以,在图形显示窗口中会出现一个白色的框,当你放开鼠标的时候,白色的框框住的部分会在窗口中全屏显示.缩小显示按住鼠标的中键,向左拖动,向上向下都可以,在图形显示窗口中会出现一个白色的框,当你放开鼠标的时候,图形缩小的程度与白色框的大小成反比.新的视图会以白色框的中心为中心.第二步:模型1.保留缺省的求解器设定Define-Models-Solver…a保留所有缺省设置.b点击OK关闭Solver面板.2.打开k-ε湍流模型Define-Models-Viscous…a在Model选单里选择k-epsilon选项,单击单选按钮或者单击文字就可以了,单击了以后,会在当选按钮中出现一个黑点.然后Viscous Model面板会展开来.b在k-epsilon Model选单中选择Realizable.c单击OK关闭Viscous Model面板.3.启动能量方程以计算传热Define-Models-energy…a单击复选框或者文本来选择Energy Equation选项.Note:当选项被选择了之后复选框中会有一个勾,如果没有就是没有选择.b单击OK关闭Energy面板.第三步:材料1.创建一种新材料叫做water.Define-Materials…a双击Name下面的输入框用鼠标来输入water作为材料名称.b按照下表在Properties属性框中输入下列数据:c单击Change/Create.一个question对话框出现了,询问你是否要覆盖空气的属性,单击No,则新材料water会被添加到材料表中去了,原来的那个选单中只有air一种材料.另外:你也可以从材料库中复制water-liquid[h2o]的物理性质单击Fluent Database…按钮就可以打开材料库了.如果材料库中的物质的物理性质跟你计算要用到的不一样,你可以在Preperties 的选框中更改它们,然后点击Change/Creat按钮来更新你的当地复制数据数据库的原始数据是不会被改变的.d打开Fluent Fluid Materials的下拉菜单确定有两种定义了的材料了.e关闭Materials面板.第四步:边界条件define-boundary conditions…1.为流体设定边界条件fluid.a在Zone选单中选择fluid选项.b单击Set…来打开Fluid面板.i.从Material Name的下拉菜单中选择water.ii.单击OK关闭Fluid面板.你刚才的操作指定了water作为本次仿真中的工作流体.2.为冷液体入口设定边界条件velocity-inlet-5提示:如果你不确定是哪个入口区域对应冷流体入口,那么你可以按照上一步说到的打开网格显示图用鼠标右键来显示这部分的特征.不光这部分区域的信息会被显示在FLUENT控制窗口中,而且会在Boundary Conditions面板中被选中.a在Zone选单中选择velocity-inlet-5.b单击Set…来打开Velocity Inlet面板.i.在Velocity Specification Method的下拉菜单中选择Components选项.Velocity Inlet面板会展开来.ii.在X-Velociyt中输入0.4m/s.iii.保留Y-Velociy和Z-Velocity的缺省值0m/s.iv.在Specification Method的下拉菜单中选择Intensity an Hydraulic Diameter湍流强度和水力直径选项.v.在Turbulent Intensity湍流强度中输入5%.vi在Hydraulic Diameter水力直径中输入4英尺.水力直径Dh的定义如下Dh上式中A是横截面积,Pw是湿周.vii.单击Thermal标签.viii.在Temperature温度栏中输入293.15Kix.单击OK关闭Velocity Inlet面板.3.用下表中的数据来设定热流体入口的边界条件:4.为出口pressure-outlet-7设定边界条件如下图中所示.Note:FLUENT只有在流体流出了出口,才会在计算区域计算回流条件.因为回流有可能在解方程的程序中出现,你需要设定合理的回流条件以防影响到计算的收敛.5.对于管壁wall保留缺省的Thermal标签中的Heat Flux的值0w/m2.6.关闭Boundary Conditions面板.第五步:求解1.初始化流场,在边界条件设定中采用冷流体入口velocity-inlet-5作为初始点.Solve-Initialize-Initialize…a从Compute From的下拉菜单中选择velocity-inlet-5.b在Initial Values选框中输入1.2m/s作为y轴的速度Y Velocity.Note: While an initial X Velocity is an appropriate guess for the horizontalsection, the addition of a Y Velocity component will give rise to a betterinitial guess throughout the entire elbow.c单击Init关闭Solution Initialization方程初始化面板.2.在计算过程中显示残差示意图.Solve-Monitors-Residual…a在Options选框中选择Plot.b如上图所示在Absolute Criteria of continuity绝对标准的连续性一栏中填入1e-05.c单击OK关闭Residual Monitors残差控制面板.Note:缺省状况下,作为FLUENT的一种判定方程收敛性的方法,所有的变量都会被监控和检查.虽然残差对于判定是否收敛很重要,但是一种更加可信的方法是定义表面监控.你将在下一步进行这一项工作.3.在出口处定义一个表面监控pressure-outlet-7.Solve-Monitors-Sur face…a单击向上的箭头的按钮把Surface Monitors设定为1.b启动monitor-1的Plot和Write选项.c把monitor-1的Every值设定为3.这一项操作是指示FLUENT在解方程过程中每三次迭代过后就更新表面监控的图形和将数据写入到文件中去.d单击Define按钮打开Define Surface Monitor面板.i.在Report Type的下拉菜单中选择Mass-Weighted Average 选项.ii. File Name一栏中保留monitor-1.out作为文件名称.iii.从 Report的下拉菜单中选择Temperature和Static Temperature.iv. 从Surfaces选单中选择pressure-outlet-7v.单击OK关闭Define Surface Monitor面板.e单击OK关闭Surface Monitors面板.4.保存算例文件elbow1.cas.gz.File-Write-Case…a可选步骤显示你想要将文件保存的目标文件夹.缺省设置中,文件会被保存在你读取elbow.msh的那个文件夹中比如说introduction文件夹.你可以改变储存路径或者新建一个文件夹来放置此文件.b在Case File中输入elbow1.cas.gz.在文件名后面加上.gz的后缀是指示FLUENT将这个文件用压缩格式保存.你不需要在文件的扩展名中写.cas比如说,如果你写的文件名是elbow1.gz,FLUENT会自动的把它保存成elbow1.cas.gz..gz这个后缀也可以用来保存数据文件为压缩格式.c确定Write Binary Files选项是选择了的,这样文件就可以保存为二进制的了.d单击OK关闭Select File对话框.Note:如果你在Select File对话框中还是使用的缺省的introduction文件夹,一个警告对话框会出现,提示你elbow1.cas.gz文件已经存在了.因为你在这个教程中所有的需要保存的文件在introduction文件夹中都是存在的,而且可以被覆盖,单击OK关掉警告对话框.5.开始计算,迭代150次.Solve-Iterate…a 在Number of Iterations迭代的次数一栏中输入150.b单击Iterate.Note:当开始计算以后,同样也开始了以在Surface Monitors面板中设定的频率来保存表面控制数据了.如果你在你的工作文件夹中出现了与你在Define Surface Monitor面板中设定的文件名同名的文件,一个Question问题对话框就会出现,询问你是否要将产生的新数据添加到已经存在的文件中去,单击NO关闭Question对话框,然后单击OK关掉警告对话框,这样FLUENT会将产生的产生的数据文件覆盖已经存在文件.当计算进行时,残差控制图会在一个图形窗口显示出来图1.3.还有一个图形窗口会显示收敛过程中的温度的加权平均值图1.4.计算会在迭代了大约140步之后达到收敛.Note:使用的平台不同,收敛所需要的次数是不一样的.而且,不同的电脑残差的值也不一样,你的电脑上出现的图像可能跟本文中的图不是完全一样的.c当计算结束了以后,关闭Iterate面板.6.检查收敛的图像图1.3和图1.4.Note:判断收敛并没有统一的标准,残差的定义对于一类的问题可能是很有用的,但对于其他种类的问题,残差反而可能会误导你.因此不光检查残差水平并且也参照相关的综合指标、质量能量平衡是判断计算收敛的一个好方法.当我们要判断计算是否收敛,有如下三个指标:残差已经下降到足够的程度了.如果每一个变量的Convergence Criterion收敛准则都达到了,那么计算就收敛了.缺省设置中,收敛准则是每一个变量的残差必须小于10-3,除了能量的残差,这一项的缺省设置是10-6.解不再随着迭代次数的增加而变化有的时候残差并不能降到你设定的值以下,但是在迭代过程中检测具有代表性的流动变量可能会发现残差已经达到一个稳定的状态,并且不会随着迭代的次数增加而改变了,这一情况也可以被视为已经收敛了.总的质量、动量、能量和数量已经达到平衡状态你可以在Flux Reports面板中检查总的质量、动量、能量和数量的平衡.计算达到收敛后,整个区域的净流量的不平衡率必须小于0.2%.下一步中,你就会学会如何从质量平衡来判断计算是否收敛.7.检查收敛的质量流量报告Report-Fluxes…a 从Boundaries的选单中选取pressure-outlet-7, velocity-inlet-5和 velocity-inlet-6.b单击 Compute.入口的流量应该跟出口的流量十分的接近,它们之间的差异会在右下角的区域以kg/s表示出来,在控制面板中也有.你可以看到,不平衡率显然要小于上文中提到的标准0.2%.c关闭Flux Reports面板.8.保存数据文件elbow1.dat.gz.File-Write-Data…在教程接下来的步骤里面,你要使用不同的前缀保存额外的算例和数据文件.第六步:展示初步解决方案1.在对称平面上展示填充了的等速度面图1.5Display-Contours…a 在Options组框里启动Filled选项.b确定Options组框里Node Values是已经启动了的.c在Contours of的下来选单中选择Velocity 和Velocity Magnitude.d在Surfaces选单中选择symmetry选项.e单击Display剖面图就会出现在图形窗口中了.另外:你在展示区域中的任一点用鼠标右击一下,这一点相应的等高线的值会在FLUENT面板中显示.2.在对称平面上展示填充了的等温面图1.5Display-Contours…a在Contours of的下拉菜单中选择Temperature和Static Temperature.b 单击Display 关闭 Contours 面板.3. 在对称平面上展示速度矢量图图1.7和图1.8Display-Vectors…a从Surfaces选单中选择symmetry.b单击Display来展示速度矢量图 Note:在Options组框中Auto Scale选项是缺省设置的,这个有时会造成矢量在大部分区域太大或者太小.c在Scale一栏中填4来增加矢量的大小.d把Skip设定为2来让矢量更适合观看.e再一次单击Display图1.7f缩放显示的矢量图.按住鼠标中键,向右边拖动向上向下都可以,屏幕中会出现一个白框,确定白框中的东西是你想要放大看得,松开鼠标,白框中的图像就被放大显示了图1.8.g缩放回到原来的视图中.按住鼠标中键向左拖动,向上向下都可以,一个白色的框就会出现在屏幕上,确定这个框的大小和你刚才放大图像的框的大小是一样的,然后松开鼠标就可以了.图像又会像图1.7那样显示了.如果在图形窗口中没有显示,你可以在靠近图形窗口的中键某处点击一下鼠标中键,然后图像就会以那一点为中心显示出来了.或者你可以在Views面板中选择初始的视图.在Views选单中选择front然后单击Apply就可以了,如下图所示.Display-Views…h关闭Vectors面板.4.在出口的中心线上创建一个线表面.不知道怎么翻译,这个面的作用是,展示出口中心线上的温度随着坐标的变化.Surface-Iso-Surfacea在Surface of Constant的下拉菜单中选择Grid 和Z-Coordinateb点击Compute.值的最大和最小值会在z方向上以最大和最小区域来显示.c在Iso-Values一栏中保存缺省设置的0英尺.d在From Surface选单中选择pressure-outlet-7一项.e在New Surface Name中输入z0 outlet.f单击Create.在line surface z0 outlet创建好了以后,一个新的New Surface Name输入给你准备好了,以备你要生成新的面.g关闭Iso-Surface面板.5.展示并保存初计算后一个在XY面上的通过出口中心线的温度剖面图.Plot-XY Plot…a在Y Axis Function的下拉菜单中选择Temperature 和Static Temperatureb在Surfaces选单中选择z0 outletc单击Plotd在Options组框中启动Write to File那么原来标记着Plot的按钮会变成Writee单击Write打开Select File对话框.i.输入outlet temp1.xy作为XY File.ii.单击OK保存温度数据,然后关闭Select File对话框f关闭Solution XY Plot面板6.为热能头方程定义一个用户自定义方程就是原来display 不能展示的,自定义一个方程让它来展示Define-Custom Field Functions…a从Field Functions的下拉菜单中选择Density 和Density,然后单击Select按钮,来讲density添加到Definition区域.b单击X按钮,将乘法符号添加到Definition区域.c从Field Functions的下拉菜单中选择Velocity 和Velocity Magnitude,然后单击Select按钮将|V|添加到Definition 定义区域.d单击y^x 表示这个量的几次方,输入2e单击/按钮,将除号添加到Definition区域,然后点击2.f输入dynamic-head作为New Function Name新功能的名字.g单击Define关闭Custom Field Function Calculator面板.7.展示填充了的用户自定义量的等高线图图1.10Display-Contours…a从Contours of的下拉菜单中选择Custom Field Functions 和dynamic-head.提示:Custom Field Functions在Contours of下拉菜单的顶部的上层,当你打开下拉菜单之后,点击右边的滚动条的向上的箭头来将菜单向上滚动.b要确定Surfaces选单中的symmetry选项是已经选中了的.c单击Display关闭Contours面板.Note:如果你上一步缩放完了没有回到全屏视野的话,这一步你可能需要缩放.8.保存算例和数据文件来保存用户自定义功能的设置elbow1.cas.gz and elbow1.dat.gz.File-Write-Case & Dataa确认你是将elbow1.cas.gz输入作为Case/Data File文件名 Note:如果你同时保存算例和数据文件,那么不论你文件的扩展名是.cas或.dat,两种文件都会被保存的.b单击OK关闭Select File对话框.第七步:启动二阶离散本教程中的前一部分解方程用的是一阶离散.这个结果是很扩散的,混合超过了预测,可以在温度和速度的分布图中看出.现在你需要使用二阶离散来使结果更加的精确.为了使用二阶离散,你需要在方程的梯度选项中将以单元为基础的改成以节点为基础的来优化能量的收敛.1.改变求解的设置Define-Models-Solver…a在Gradient Option的选单中选择Green-Gauss Node Based Note:这个选项在非结构性网格的计算上比以单元为基础的计算方法更好,它可以提供更好的能量收敛.b单击OK关闭Solver面板.2.使用二阶计划计算所列出的方程Solve-Controls-Solution…a在Under-Relaxation Factors组框中保留缺省的值.b从Discretization组框中的Pressure的下拉菜单中选择Second Orderc从Momentum, Turbulent Kinetic Energy,Turbulent Dissipation Rate和 Energy的下拉菜单中选择Second Order Upwind d单击OK关闭Solution Controls面板.3.请求150的迭代来继续计算Solve-IAterate…另外:如果你要保存这一次的表面监控的收敛历史的迭代作为一个输出文件的话,你要事先在Define Surface Monitor里边把文件名改成monitor-2.out来继续计算.a确认迭代的次数Number of Iterations是150次.b单击Iterate然后当计算结束后关闭Iterate面板解会在第二次的迭代中在大约57步的时候达到收敛图1.11,收敛历史如图1.12.Note:当你改变了求解的参数的时候,残差是会有突变的.4.为二阶离散的求解保存算例和数据文件elbow2.cas.gz 和elbow2.dat.gz.File-Write-Case & Dataa 输入elbow2.gz作为 Case/Data File的名字.b 单击OK关闭Select File 对话框.文件elbow2.cas.gz和elbow2.dat.gz会在你的文件夹中被保存.5.检查修正后的等温面图1.13Display-Contours…a确定在Options组框中Filled是选中了的.b从Contours of的下拉菜单中选择Temperature 和Static Temperature.c确认Surfaces选单中symmetry是选中了的.d单击Display关闭Contours面板.图1.13显示,在弯头的外壁侧,温流体的热量传递要更加快一点.比较图1.13和图1.6来看一看二阶离散的效果.6. 展示并保存二阶计算后一个在XY面上的通过出口中心线的温度剖面图图1.14.Plot-XY Plot…。
fluent燃烧说明介绍
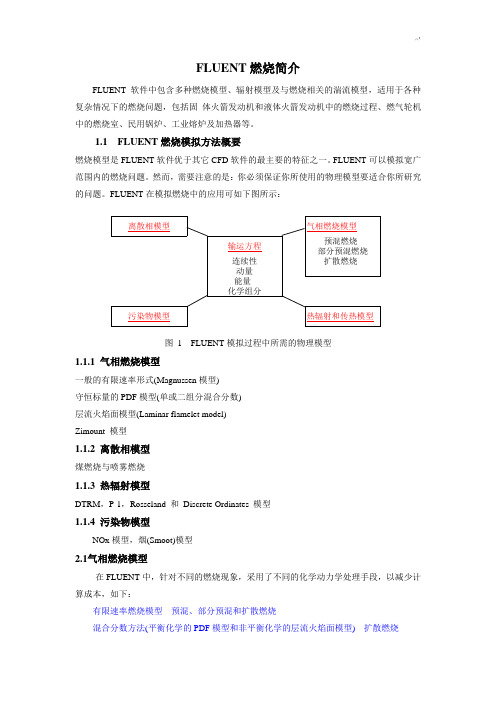
FLUENT燃烧简介FLUENT软件中包含多种燃烧模型、辐射模型及与燃烧相关的湍流模型,适用于各种复杂情况下的燃烧问题,包括固体火箭发动机和液体火箭发动机中的燃烧过程、燃气轮机中的燃烧室、民用锅炉、工业熔炉及加热器等。
1.1 FLUENT燃烧模拟方法概要燃烧模型是FLUENT软件优于其它CFD软件的最主要的特征之一。
FLUENT可以模拟宽广范围内的燃烧问题。
然而,需要注意的是:你必须保证你所使用的物理模型要适合你所研究的问题。
FLUENT在模拟燃烧中的应用可如下图所示:图 1 FLUENT模拟过程中所需的物理模型1.1.1 气相燃烧模型一般的有限速率形式(Magnussen模型)守恒标量的PDF模型(单或二组分混合分数)层流火焰面模型(Laminar flamelet model)Zimount 模型1.1.2 离散相模型煤燃烧与喷雾燃烧1.1.3 热辐射模型DTRM,P-1,Rosseland 和Discrete Ordinates 模型1.1.4 污染物模型NOx模型,烟(Smoot)模型2.1气相燃烧模型·在FLUENT中,针对不同的燃烧现象,采用了不同的化学动力学处理手段,以减少计算成本,如下:有限速率燃烧模型---预混、部分预混和扩散燃烧混合分数方法(平衡化学的PDF模型和非平衡化学的层流火焰面模型)---扩散燃烧反应进度方法(Zimont模型)---预混燃烧混合物分数和反应进度方法的结合---部分预混燃烧2.2.1 有限速率模型化学反应过程一般采用总包机理(即简化化学反应,如单步反应)进行描述。
求解积分的输运方程,得到每种组分的时均质量分数值,如下:-----(1)其中组分j的反应源项为所有反应K个反应中,组分j的净生成速率:-----(2)-----(3)计算所需参数包括:1、组分及其热力学参数值;2、反应及其速率常数值。
有限速率模型的有缺点:优点:适用于预混、部分预混和扩散燃烧,简单直观;缺点:当混合时间尺度和反应时间尺度相当时缺乏真实性,难以解决化学反应与湍流的耦合问题,难以预测反应的中间组分,模型常数具有不确定性。
基于Fluent的燃料电池建模及仿真研究

基于Fluent的燃料电池建模及仿真研究郭苗苗;文陈;陈学成;陈永刚【摘要】宇航用质子交换膜燃料电池在微重力环境下的流动特性是影响其工作性能和使用寿命的重要因素,对其本身设计与优化也有着十分重要的意义.针对质子交换膜燃料电池常用流道,基于流体仿真软件Fluent,建立三维流体仿真模型,采用计算流体动力学数值方法,对其在微重力条件下的流动特性和分布特点进行了仿真研究.研究表明,流体流动与传质有着密切关系,对伏安输出特性曲线起到决定性作用,研究结果可以为宇航用质子交换膜燃料电池的设计改进提供依据.【期刊名称】《电源技术》【年(卷),期】2019(043)001【总页数】4页(P113-116)【关键词】质子交换膜燃料电池;计算流体动力学;建模【作者】郭苗苗;文陈;陈学成;陈永刚【作者单位】中国空间技术研究院北京卫星制造厂,北京100094;中国空间技术研究院北京卫星制造厂,北京100094;中国空间技术研究院北京卫星制造厂,北京100094;中国空间技术研究院北京卫星制造厂,北京100094【正文语种】中文【中图分类】TM911.4随着空间技术和信息技术的飞速发展,新的航天任务对电源系统提出了高功率、长寿命、服役稳定等需求。
但由于受质量、体积、无污染、新的飞行工况、可靠性与安全性等的严格限制,目前我国采用的太阳电池、蓄电池及PCU集成储能发电系统已经不能适用于一些承担特殊任务的航天器。
质子交换膜燃料电池(PEMFC)直接通过氢氧电化学反应把化学能转换成电能,其效率通常高于其它发电装置,在反应过程中不涉及到燃烧,可以长时间不间断地工作,同时兼具普通化学电源能转换效率高和常规发电机组连续工作时间长两种优势[1-3]。
燃料电池流道仿真设计一直是燃料电池研究的热点[4-10]。
简等[7]研究了不同孔隙率对流道内气体洼速和沿流道方向的压力分布情况,以电池输出电流密度为参考标准,研究孔隙率对电池输出性能的影响。
fluent 实用资料
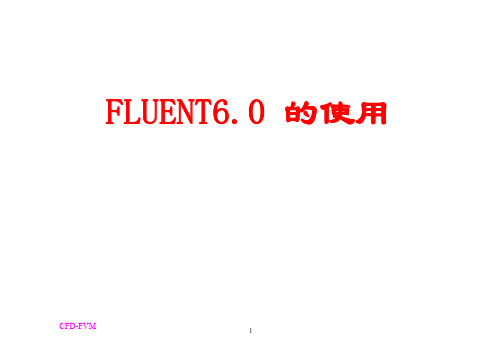
E E E
S
S
S
C
C
C
R
R
CFD-FVM
13
�
Formula for map scheme:
4*End+N*Side
�
Formula for submap scheme:
4*End+ L*Side + M*(E+C) + N* (2*E+R)
E
E
S
S
C E E
C E E E
S E
S E E
Default
�
对于二维图形来说,它必须是一个 region,也就 是说要求是一个联通域。 对于三维图形而言,要求其是一个 ASCI body
�
CFD-FVM
18
�
由于各软件设置的最小识别尺寸不同, 导入后的几何体可能会出现:
� �
不完整、有缝隙的几何体 有一些CFD分析时不需要的一些细小的几何结构
�
清理过程主要采用 gambit中的虚几何操作。
�
CFD-FVM
16
策略
� � � �
�
Boundary layers Pre-meshing Sizing functions 为降低离散误差,减 少单元数量,最好使 用hex(六面体网格) 对形状复杂的几何体 可分解成几个简单几 何体再用六面体网格
CFD-FVM
17
� � �
Gambit可读入其它CFD软件生成的图形 也可读入autocad proE等cad软件生成的图形 CAD中创建的图形要输出为.sat文件,要满 足一定的条件。
CFD-FVM
12
顶点类型
- 1、下载文档前请自行甄别文档内容的完整性,平台不提供额外的编辑、内容补充、找答案等附加服务。
- 2、"仅部分预览"的文档,不可在线预览部分如存在完整性等问题,可反馈申请退款(可完整预览的文档不适用该条件!)。
- 3、如文档侵犯您的权益,请联系客服反馈,我们会尽快为您处理(人工客服工作时间:9:00-18:30)。
∂T ∂x
2
∂T + ∂y
2
∂T + ∂z
2
Technical Support
If you encounter difficulties while using FLUENT, please first refer to the section(s) of the manual containing information on the commands you are trying to use or the type of problem you are trying to solve. The product documentation is available from the online help on the documentation CD, or from the Fluent Inc. User Services Center (). If you encounter an error, please write down the exact error message that appeared and note as much information as you can about what you were doing in FLUENT. Then refer to the following resources available on the Fluent Inc. User Services Center (): • Installation and System FAQs - link available from the main page on the User Services Center. The FAQs can be searched by word or phrase, and are available for general installation questions as well as for products. • Known defects for FLUENT - link available from the product page. The defects can be searched by word or phrase, and are listed by categories. • Online Technical Support - link available from the main page on the User Services Center. From the Online Technical Support Portal page, there is a link to the Search Solutions & Request Support page, where the solutions can be searched by word or phrase. The User Services Center also provides online forums, where you can discuss topics of mutual interest and share ideas and information with other Fluent users, and the ability to sign up for e-mail notifications on our latest product releases.
i
) marks an important note.
! ) marks a warning.
• Different type styles are used to indicate graphical user interface menu items and text interface menu items (e.g., Iso-Surface panel, surface/iso-surface command). • The text interface type style is also used when illustrating exactly what appears on the screen or exactly what you need to type into a field in a panel. The information displayed on the screen is enclosed in a large box to distinguish it from the narrative text, and user inputs are often enclosed in smaller boxes. • A mini flow chart is used to indicate the menu selections that lead you to a specific command or panel. For example, Define −→Boundary Conditions... indicates that the Boundary Conditions... menu item can be selected from the Define pull-down menu, and display −→grid indicates that the grid command is available in the display text menu. The words before the arrows invoke menus (or submenus) and the arrows point from a specific menu toward the item you should select from that menu. In this
This tensor is usually written as
∂vx ∂x ∂vy ∂x ∂vz ∂x ∂vx ∂y ∂vy ∂y ∂vz ∂y ∂vx ∂z ∂vy ∂z ∂vz ∂z
UTM-2
c Fluent Inc. January 4, 2005
– The gradient of a vector quantity is a second-order tensor; for example, in Cartesian coordinates, ∇(v ) = ∂ ∂ ∂ ı+ + k ∂x ∂y ∂z vx ı + vy + vz k
Fluent Inc. Centerra Resource Park 10 Cavendish Court Lebanon, NH 03766
Using This Manual
What’s In This Manual
The FLUENT Fuel Cell Modules Manual tells you what you need to know to model polymer electrolyte membrane (PEM) fuel cells or solid oxide fuel cell (SOFC) fuel cells with FLUENT. In this manual, you will find background information pertaining to the models, a theoretical discussion of the models used in FLUENT, and a description of using the models for your CFD simulations.
Using This Manual
– The divergence of a vector quantity, which is the inner product between ∇ and a vector; for example, ∇·v = ∂vx ∂vy ∂vz + + ∂x ∂y ∂z
– The operator ∇ · ∇, which is usually written as ∇2 and is known as the Laplacian; for example, ∇2 T = ∂2T ∂2T ∂2T + + ∂x2 ∂y 2 ∂z 2
∇2 T is different from the expression (∇T )2 , which is defined as (∇T ) =
Typographical Conventions
Several typographical conventions are used in this manual’s text to facilitate your learning process.
• An informational icon ( • An warning icon (
Airpak, FIDAP, FLUENT, FloWizard, GAMBIT, Icemax, Icepak, Icepro, MixSim, and POLYFLOW are registered trademarks of Fluent Inc. All other products or name brands are trademarks of their respective holders. CHEMKIN is a registered trademark of Reaction Design Inc. Portions of this program include material copyrighted by PathScale Corporation 2003-2004.
c Fluent Inc. January ng This Manual