三甘醇再生
三甘醇再生方法
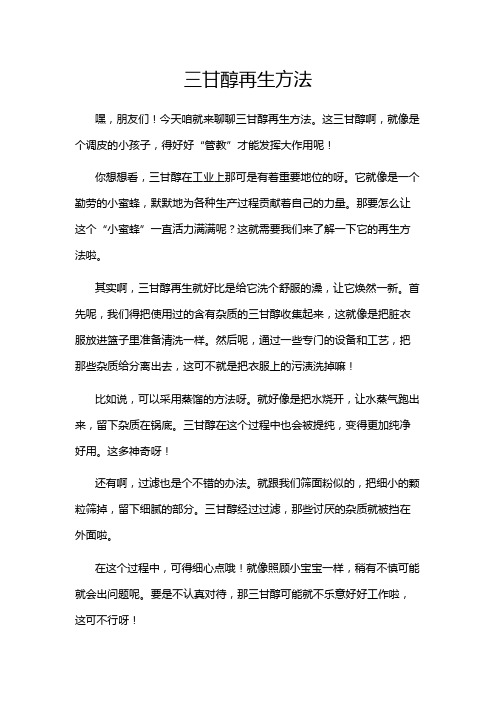
三甘醇再生方法嘿,朋友们!今天咱就来聊聊三甘醇再生方法。
这三甘醇啊,就像是个调皮的小孩子,得好好“管教”才能发挥大作用呢!你想想看,三甘醇在工业上那可是有着重要地位的呀。
它就像是一个勤劳的小蜜蜂,默默地为各种生产过程贡献着自己的力量。
那要怎么让这个“小蜜蜂”一直活力满满呢?这就需要我们来了解一下它的再生方法啦。
其实啊,三甘醇再生就好比是给它洗个舒服的澡,让它焕然一新。
首先呢,我们得把使用过的含有杂质的三甘醇收集起来,这就像是把脏衣服放进篮子里准备清洗一样。
然后呢,通过一些专门的设备和工艺,把那些杂质给分离出去,这可不就是把衣服上的污渍洗掉嘛!比如说,可以采用蒸馏的方法呀。
就好像是把水烧开,让水蒸气跑出来,留下杂质在锅底。
三甘醇在这个过程中也会被提纯,变得更加纯净好用。
这多神奇呀!还有啊,过滤也是个不错的办法。
就跟我们筛面粉似的,把细小的颗粒筛掉,留下细腻的部分。
三甘醇经过过滤,那些讨厌的杂质就被挡在外面啦。
在这个过程中,可得细心点哦!就像照顾小宝宝一样,稍有不慎可能就会出问题呢。
要是不认真对待,那三甘醇可能就不乐意好好工作啦,这可不行呀!而且哦,不同的情况可能需要不同的再生方法呢。
这就跟我们穿衣服一样,不同的场合要穿不同的衣服,总不能穿着睡衣去上班吧!所以呀,要根据实际情况灵活选择再生方法。
大家想想,要是没有好的三甘醇再生方法,那得浪费多少资源呀!那可都是钱呀,咱能眼睁睁看着它们溜走吗?当然不能啦!所以呀,一定要重视这个三甘醇再生,让它能一直为我们服务。
总之呢,三甘醇再生方法是非常重要的,我们可不能小瞧它。
只有把这个“小调皮”管好了,它才能乖乖地为我们创造价值呀!大家说是不是这个理儿呢?。
三甘醇再生系统
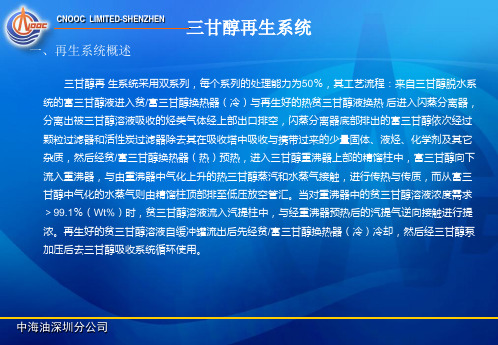
三、主要设备描述
1、闪蒸罐
(1)简述 三甘醇闪蒸罐为立式两相分离器,主要用于分离从富甘醇中闪蒸出的气体。闪蒸出的气体从罐顶排
出,富甘醇从底部排放到下游滤器,少量的油积聚在表层并溢流进入罐内的一个油槽,由手动排放 阀排放到闭排系统。
(2)主要设计参数
设备名称 用途 尺寸(mm) 设计压力(KPaG) 设计温度(℃)
操作压力(KPaG) 操作温度
三甘醇闪蒸罐(DPP-V-2220A/B)
闪蒸三甘醇中的天然气
610(OD)×2286(S/S)
1655 -29~120 340 62.66
中海油深圳分公司
CNOOC LIMITED-SHENZHEN
(3)仪表监测及关断(以A套为例)
①压力控制和监测
闪蒸罐的操作压力通过气体进口、出口的2个压力控制阀来稳定,并由容器上的压力表PI-2210现场显示。如果罐内的 压力高于340KPaG将开大PCV-2201;如果压力不足300KPaG将开大PCV-2204,由低压燃料系统的气体来提高罐内压力 。当压力出现过高(400KPaG)或过低(250KPaG)时,现场压力变送器PIT-2201触动中控室报警。当压力出现高高( 600KPaG)时,气体出口管线上的高高压力变送器PIT-2202将触动中控室控制盘报警,并发出4级关断信号,同时关闭吸 收塔富甘醇出口的SDV-2105和闪蒸罐覆盖气入口的SDV-2201。另外,在闪蒸罐上还安装有压力安全阀PSV-2201A/B, 用于对罐体进行压力二级保护,当压力达到其设定值(1000KPa)时,进行压力释放。
②液位监测 三甘醇聚结器的液位通过液位计LG-2235现场指示。
6.三甘醇循环泵
(1)简述 三甘醇循环泵从三甘醇聚结器接受贫甘醇,将其加压输送至吸收塔。属于低流量高压头柱塞泵。
三甘醇损耗原因分析及常见处理办法介绍
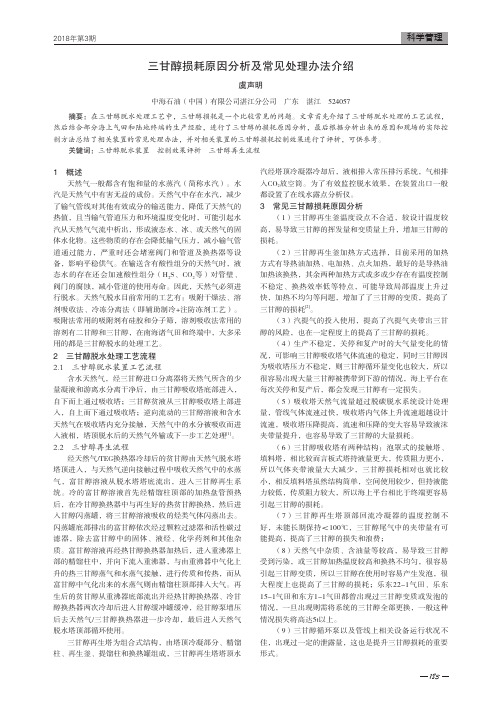
1831 概述天然气一般都含有饱和量的水蒸汽(简称水汽)。
水汽是天然气中有害无益的成份。
天然气中存在水汽,减少了输气管线对其他有效成分的输送能力,降低了天然气的热值,且当输气管道压力和环境温度变化时,可能引起水汽从天然气气流中析出,形成液态水、冰、或天然气的固体水化物。
这些物质的存在会降低输气压力,减小输气管道通过能力,严重时还会堵塞阀门和管道及换热器等设备,影响平稳供气。
在输送含有酸性组分的天然气时,液态水的存在还会加速酸性组分(H 2S、CO 2等)对管壁、阀门的腐蚀,减小管道的使用寿命。
因此,天然气必须进行脱水。
天然气脱水目前常用的工艺有:吸附干燥法、溶剂吸收法、冷冻分离法(即辅助制冷+注防冻剂工艺)。
吸附法常用的吸附剂有硅胶和分子筛,溶剂吸收法常用的溶剂有二甘醇和三甘醇,在南海诸气田和终端中,大多采用的都是三甘醇脱水的处理工艺。
2 三甘醇脱水处理工艺流程2.1 三甘醇脱水装置工艺流程含水天然气,经三甘醇进口分离器将天然气所含的少量凝液和游离水分离干净后,由三甘醇吸收塔底部进入,自下而上通过吸收塔;三甘醇贫液从三甘醇吸收塔上部进入,自上而下通过吸收塔;逆向流动的三甘醇溶液和含水天然气在吸收塔内充分接触,天然气中的水分被吸收而进入液相,塔顶脱水后的天然气外输或下一步工艺处理[1]。
2.2 三甘醇再生流程经天然气/TEG换热器冷却后的贫甘醇由天然气脱水塔塔顶进入,与天然气逆向接触过程中吸收天然气中的水蒸气,富甘醇溶液从脱水塔塔底流出,进入三甘醇再生系统。
冷的富甘醇溶液首先经精馏柱顶部的加热盘管预热后,在冷甘醇换热器中与再生好的热贫甘醇换热,然后进入甘醇闪蒸罐,将三甘醇溶液吸收的烃类气体闪蒸出去。
闪蒸罐底部排出的富甘醇依次经过颗粒过滤器和活性碳过滤器,除去富甘醇中的固体、液烃、化学药剂和其他杂质。
富甘醇溶液再经热甘醇换热器加热后,进入重沸器上部的精馏柱中,并向下流入重沸器,与由重沸器中气化上升的热三甘醇蒸气和水蒸气接触,进行传质和传热,而从富甘醇中气化出来的水蒸气则由精馏柱顶部排入大气。
某三甘醇天然气脱水工艺设计--------再生塔设计概述
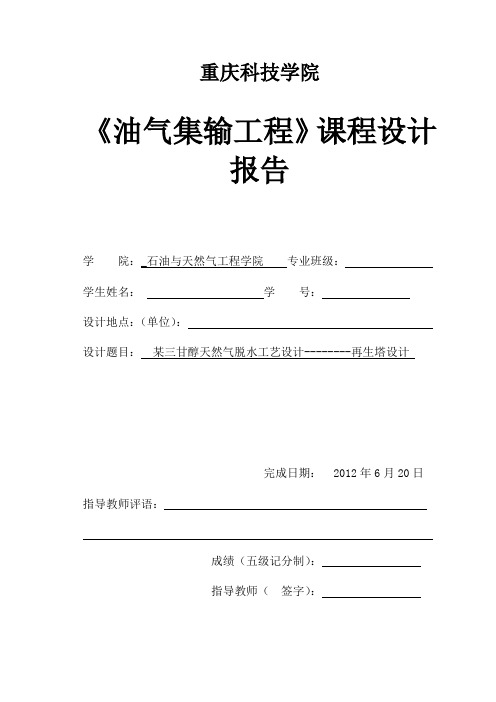
重庆科技学院《油气集输工程》课程设计报告学院:_石油与天然气工程学院专业班级:学生姓名:学号:设计地点:(单位):设计题目:某三甘醇天然气脱水工艺设计--------再生塔设计完成日期: 2012年6月20日指导教师评语:成绩(五级记分制):指导教师(签字):天然气中的水对于天然气的输送和使用都是有害的,因此,在经济条件允许的情况下,尽可能的脱去天然气中的水,不论对于天然气输送还是使用都非常的有必要。
天然气中的水通常以气态和液态两种形式存在,在少数情况下也会呈固态。
三甘醇在吸收塔中吸收了水分变成富液,不能再继续使用。
因此,再生塔就为富甘醇进行再生,并且打入吸收塔中再次利用。
三甘醇再生塔是安装在重沸器(再沸器)顶部的立式分馏塔。
通过三甘醇脱水工艺流程,TEG吸收塔底部排出的三甘醇富液与TEG再生塔顶部换热后进入TEG闪蒸罐,尽可能闪蒸出其中所溶的烃类,闪蒸后的三甘醇富液经过TEG过滤器除去固体、液体杂质,进入TEG换热罐提高三甘醇进TEG再生塔的温度,从再生塔中部进料,经TEG重沸器加热再生,再生后的三甘醇贫液经TEG换热罐和TEG后冷器冷却,冷却后的三甘醇贫液由TEG 循环泵输送到干气/贫甘醇换热器与吸收塔顶部出来的天然气换热后进入吸收塔,实现三甘醇贫液的循环利用。
由此可见三甘醇再生塔在三甘醇脱水工艺流程中显得尤为重要。
本篇就重点介绍三甘醇再生塔在脱水工艺流程中的设计和注意事项。
关键词:三甘醇再生塔精馏柱填料塔冷却盘管三甘醇贫液的循环利用1.设计参数 (3)2.遵循的规范、标准 (4)3.再生塔设计 (5)3.1再生塔工作原理 (5)3.2再生塔塔设备的选型 (5)3.3三甘醇再生方法选择 (6)3.4参数对比及方案优选 (7)4.三甘醇再生塔的计算 (9)4.1富液精馏柱计算 (10)4.2贫液精馏柱工艺计算 (11)4.3富液精馏柱顶部冷却盘管工艺计算 (11)4.4三甘醇再生塔主要设备选型计算结果 (12)5.结论 (13)6.参考文献 (14)1.设计参数基础资料:天然气组成如下表:原料气处理量 40×104m3/d原料气露点 30~36 ºC原料气压力 2.05~2.25MPa (g)拟建天然气脱水装置产品气为干净化天然气,该产品气质量符合国家标准《天然气》(GB17820-1999)中二类气的技术指标。
最新三甘醇再生系统
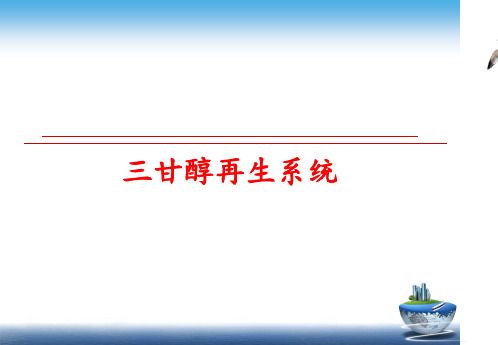
中海油深圳分公司
CNOOC LIMITED-SHENZHEN
四、正常操作需要注意的问题
1.使用正确的方法来调试和启动三甘醇脱水系统,保持再沸器的液位正常,按照需要补 充三甘醇(三甘醇的最小耗量是16g/1000Sm3gas)。
2.检查LG-2106、LG-2202,确保接触塔和闪蒸罐内没有富甘醇和烃类冷凝物存在。如 果发现烃类冷凝物聚集,应该排除。
(3)仪表监测(以A套为例) ①温度控制及监测 换热器的冷侧及热侧流体温度分别通过温度计TI-2210、2210A及TI-2211、2209现场指示。当富甘 醇侧温度出现异常时,可通过温度变送器TIT-2238触动中控盘报警。
②压力监测 富甘醇进出口压力由PI-2208及PI-2205现场指示
③安全特征 在富甘醇管线上设有安全阀PSV-2238,设定值是1000KPaG,以防管线超压。
7.闪蒸出的来的水蒸汽流出再沸器的精馏柱后,应该尽快从装置中排出。透气管线应该 有少量的液滴,以避免冷凝的水蒸汽回到装置中。
8.系统单元关断且再沸器的温度大大降低时,在循环启动前再沸器应该达到操作温度。 中海油深圳分公司
TEG PRRICULATE FILTER B
TEG PRRICULATE FILTER A
TEG CARBON FILTER
LEAN/RICH HEAT EXCRANGER
中海油深圳分公司
TEG ACCUMULATOR
TEG CIRCULATION PUMP A1/A2
CNOOC LIMITED-SHENZHEN
中海油深圳分公司
CNOOC LIMITED-SHENZHEN
③压力控制及监测 再沸器的操作压力是依靠顶部放空气体的回压来决定,由压力表PI-2202现场显示。压力过高(30KPaG)时, 变送器PIT-2215传回中控室进行报警;压力高高(170KPaG)时,关断SDV-2202。回流冷凝器上也设有温度表 PI-2203进行现场显示。此外,再沸器上还设有压力安全阀PSV-2213A/B进行压力保护。
某三甘醇天然气脱水工艺设计--------再生塔设计
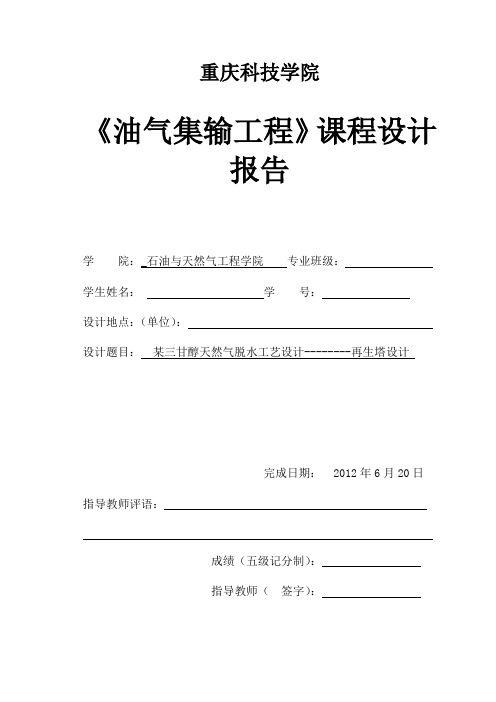
重庆科技学院《油气集输工程》课程设计报告学院:_石油与天然气工程学院专业班级:学生姓名:学号:设计地点:(单位):设计题目:某三甘醇天然气脱水工艺设计--------再生塔设计完成日期: 2012年6月20日指导教师评语:成绩(五级记分制):指导教师(签字):天然气中的水对于天然气的输送和使用都是有害的,因此,在经济条件允许的情况下,尽可能的脱去天然气中的水,不论对于天然气输送还是使用都非常的有必要。
天然气中的水通常以气态和液态两种形式存在,在少数情况下也会呈固态。
三甘醇在吸收塔中吸收了水分变成富液,不能再继续使用。
因此,再生塔就为富甘醇进行再生,并且打入吸收塔中再次利用。
三甘醇再生塔是安装在重沸器(再沸器)顶部的立式分馏塔。
通过三甘醇脱水工艺流程,TEG吸收塔底部排出的三甘醇富液与TEG再生塔顶部换热后进入TEG闪蒸罐,尽可能闪蒸出其中所溶的烃类,闪蒸后的三甘醇富液经过TEG过滤器除去固体、液体杂质,进入TEG换热罐提高三甘醇进TEG再生塔的温度,从再生塔中部进料,经TEG重沸器加热再生,再生后的三甘醇贫液经TEG换热罐和TEG后冷器冷却,冷却后的三甘醇贫液由TEG 循环泵输送到干气/贫甘醇换热器与吸收塔顶部出来的天然气换热后进入吸收塔,实现三甘醇贫液的循环利用。
由此可见三甘醇再生塔在三甘醇脱水工艺流程中显得尤为重要。
本篇就重点介绍三甘醇再生塔在脱水工艺流程中的设计和注意事项。
关键词:三甘醇再生塔精馏柱填料塔冷却盘管三甘醇贫液的循环利用1.设计参数 (3)2.遵循的规范、标准 (4)3.再生塔设计 (5)3.1再生塔工作原理 (5)3.2再生塔塔设备的选型 (5)3.3三甘醇再生方法选择 (6)3.4参数对比及方案优选 (7)4.三甘醇再生塔的计算 (9)4.1富液精馏柱计算 (10)4.2贫液精馏柱工艺计算 (11)4.3富液精馏柱顶部冷却盘管工艺计算 (11)4.4三甘醇再生塔主要设备选型计算结果 (12)5.结论 (13)6.参考文献 (14)1.设计参数基础资料:天然气组成如下表:原料气处理量 40×104m3/d原料气露点 30~36 ºC原料气压力 2.05~2.25MPa (g)拟建天然气脱水装置产品气为干净化天然气,该产品气质量符合国家标准《天然气》(GB17820-1999)中二类气的技术指标。
天然气的脱水三甘醇
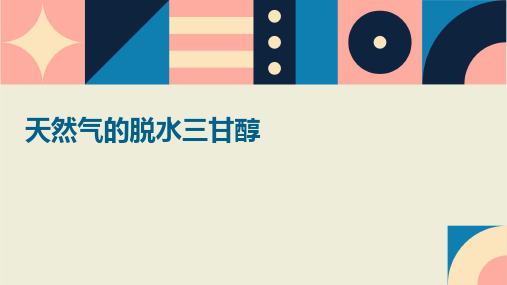
三甘醇脱水是利用其吸水性质,将天然气中的水分吸收并脱除的过程。在天然气处理过程中,三甘醇 作为脱水剂被喷洒到天然气中,与天然气充分接触,吸收其中的水分,然后通过分离器将吸收了水分 的三甘醇与天然气分离,从而达到脱水的目的。
三甘醇脱水工艺流程
预处理
首先,对天然气进行预处理,去除其中的杂质和 固体颗粒,以免对后续设备和管道造成堵塞或损 坏。
分离器
分离器用于将吸收了水分的三甘醇与天然气分离。分离器应具有合理的结构和尺寸,以确 保三甘醇和天然气的有效分离,并减少三甘醇的夹带损失。
加热器
加热器用于对吸收了水分的三甘醇进行加热再生。加热器应具有足够的加热功率和温度控 制精度,以确保三甘醇中的水分被完全蒸发掉,同时避免过高的温度对三甘醇造成热分解 或氧化等不良影响。
余热回收技术
对脱水过程中产生的余热进行回收利用,如用于加热原料气或生 产热水等,以减少能源消耗。
智能化控制技术
应用智能化控制技术对脱水过程进行实时监控和优化控制,提高 生产效率和能源利用效率。
THANKS
感谢观看
03
三甘醇脱水系统操作与维护
系统启动与停止操作
启动前准备
检查系统各部件是否完好,确认 电源、气源等供应是否正常,准 备好所需工具和材料。
启动步骤
按照操作规程逐步启动系统,包 括开启进料阀、启动循环泵、调 整操作参数等。
停止操作
在停止系统前,需要先关闭进料 阀,停止循环泵,然后按照操作 规程逐步停止系统。
吸收法
利用吸湿剂吸收水分,适用于低压、 中温环境,需定期更换吸湿剂。
膜分离法
利用特殊膜材料对水分子的选择性 透过性实现脱水,适用于各种压力、 温度条件,但投资成本较高。
三甘醇脱水中再生废气的回收利用
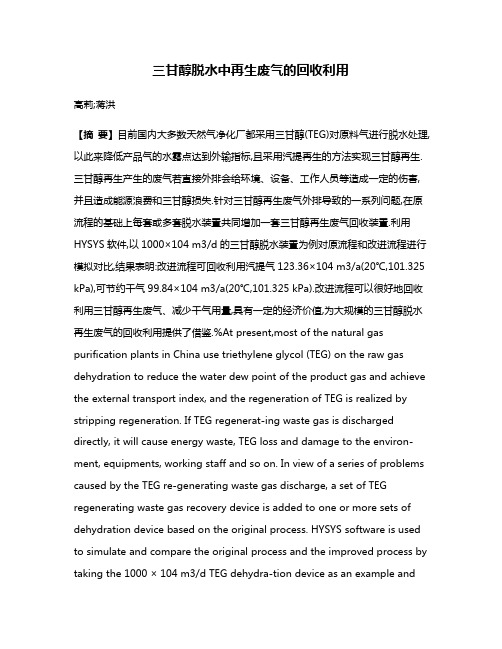
三甘醇脱水中再生废气的回收利用高莉;蒋洪【摘要】目前国内大多数天然气净化厂都采用三甘醇(TEG)对原料气进行脱水处理,以此来降低产品气的水露点达到外输指标,且采用汽提再生的方法实现三甘醇再生.三甘醇再生产生的废气若直接外排会给环境、设备、工作人员等造成一定的伤害,并且造成能源浪费和三甘醇损失.针对三甘醇再生废气外排导致的一系列问题,在原流程的基础上每套或多套脱水装置共同增加一套三甘醇再生废气回收装置.利用HYSYS软件,以1000×104 m3/d的三甘醇脱水装置为例对原流程和改进流程进行模拟对比,结果表明:改进流程可回收利用汽提气123.36×104 m3/a(20℃,101.325 kPa),可节约干气99.84×104 m3/a(20℃,101.325 kPa).改进流程可以很好地回收利用三甘醇再生废气、减少干气用量,具有一定的经济价值,为大规模的三甘醇脱水再生废气的回收利用提供了借鉴.%At present,most of the natural gas purification plants in China use triethylene glycol (TEG) on the raw gas dehydration to reduce the water dew point of the product gas and achieve the external transport index, and the regeneration of TEG is realized by stripping regeneration. If TEG regenerat-ing waste gas is discharged directly, it will cause energy waste, TEG loss and damage to the environ-ment, equipments, working staff and so on. In view of a series of problems caused by the TEG re-generating waste gas discharge, a set of TEG regenerating waste gas recovery device is added to one or more sets of dehydration device based on the original process. HYSYS software is used to simulate and compare the original process and the improved process by taking the 1000 × 104 m3/d TEG dehydra-tion device as an example andthe results show: im proved process can recycle 123.36 × 104 m3/a (20℃ ,101.325 kPa) of the TEG regenerating waste gas and save 99.84 × 104 m3/a(20℃ ,101.325 kPa) of dry gas.The improved process can better recycle the TEG regeneration waste gas and reduce the use of dry gas. What is more, it has a certain economic value and can provide reference for the recycling of TEG waste gas in large-scale TEG dehydration.【期刊名称】《油气田地面工程》【年(卷),期】2017(036)007【总页数】4页(P47-50)【关键词】三甘醇脱水;三甘醇再生废气;改进方案;HYSYS模拟;废气回收;节约干气【作者】高莉;蒋洪【作者单位】西南石油大学石油与天然气工程学院;西南石油大学石油与天然气工程学院【正文语种】中文目前,国内大多数天然气净化厂脱水装置都采用溶剂吸收法中最常用的三甘醇脱水[1-2]工艺来降低产品气的水露点,使水露点达到外输气指标。
三甘醇受污染因素及回收利用方法
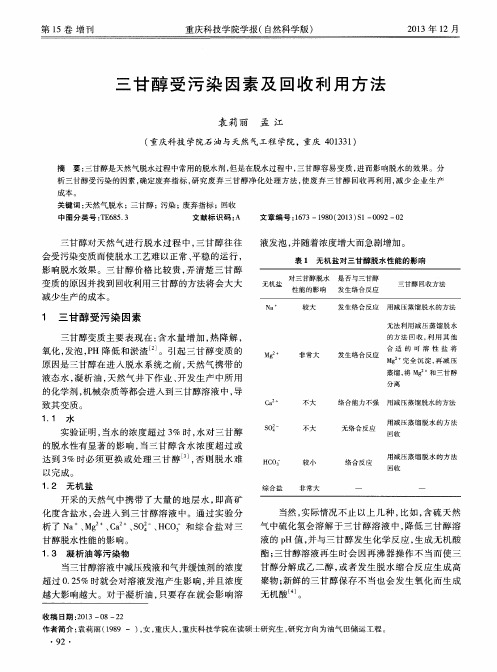
三 甘 醇 受 污 染 因 素 及 回收 利 用 方 法
袁 莉丽 孟 江
( 重庆科技 学院石 油与天 然 气工程 学院 ,重庆 4 0 1 3 3 1 )
摘 要: 三甘醇是天然气脱水过程 中常用 的脱水剂 , 但是在脱水过程 中, 三甘醇容易变 质 , 进 而影 响脱水 的效果 。分
变 质 的原 因并 找到 回收利用 三甘 醇 的方法将 会 大大
减 少生产 的成 本 。
1 三甘醇受污染因素
三 甘醇变 质 主要 表 现在 : 含 水量 增 加 , 热 降解 , 氧化 , 发泡, P H 降低 和 淤 渣 J 。引 起 三甘 醇 变 质 的
原 因是 三甘醇 在 进人 脱 水 系 统之 前 , 天然 气 携 带 的 液 态水 , 凝析油 , 天然 气 井 下作 业 、 开 发 生产 中所用 的化学剂 , 机械 杂质 等都会 进人 到三 甘醇溶 液 中 , 导 致 其变质 。
对 于三 甘醇废 弃指 标 , 目前 还没 有统 一 的标 准 , 但 主要 考 虑 3个 方 面 : 含水量低于 2 . 5 7 %, 有 机 杂
质不 大 于 0 . 3 %( 重量 ) , 含 盐量 小 于 1 0 0 0 mg / L 。
此 方法 即选 用特 定 的化 学剂 与废 三甘 醇 中 的杂 质 发生 反应 , 使 杂质 中 的离 子得 到沉 淀 , 然 后分 离 。 膜 分离 法是 最具 前 景 的方 法 , 值 得 我 们 进 一 步 深 入研 究 。
收 稿 日期 : 2 0 1 3 —0 8— 2 2
当然 , 实 际情 况 不 止 以上 几 种 , 比如 , 含 硫 天 然
气 中硫 化 氢会溶 解 于三 甘醇 溶 液 中 , 降 低 三 甘醇 溶 液的 p H值 , 并 与三 甘醇 发生 化 学 反应 , 生 成 无机 酸
友谊号三甘醇脱水及再生系统运行问题的解决
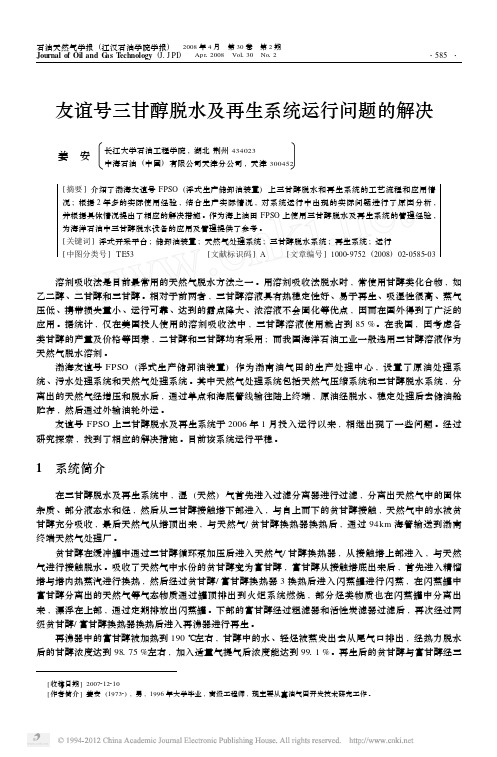
[收稿日期]2007212210 [作者简介]姜安(19732),男,1996年大学毕业,高级工程师,现主要从事油气田开发技术研究工作。
友谊号三甘醇脱水及再生系统运行问题的解决 姜 安 长江大学石油工程学院,湖北荆州434023中海石油(中国)有限公司天津分公司,天津300452[摘要]介绍了渤海友谊号FPSO (浮式生产储卸油装置)上三甘醇脱水和再生系统的工艺流程和应用情况;根据2年多的实际使用经验,结合生产实际情况,对系统运行中出现的实际问题进行了原因分析,并根据具体情况提出了相应的解决措施。
作为海上油田FPSO 上使用三甘醇脱水及再生系统的管理经验,为海洋石油中三甘醇脱水设备的应用及管理提供了参考。
[关键词]浮式开采平台;储卸油装置;天然气处理系统;三甘醇脱水系统;再生系统;运行[中图分类号]TE53[文献标识码]A [文章编号]100029752(2008)022*******溶剂吸收法是目前最常用的天然气脱水方法之一。
用溶剂吸收法脱水时,常使用甘醇类化合物,如乙二醇、二甘醇和三甘醇。
相对于前两者,三甘醇溶液具有热稳定性好、易于再生、吸湿性很高、蒸气压低、携带损失量小、运行可靠、达到的露点降大、浓溶液不会固化等优点,因而在国外得到了广泛的应用。
据统计,仅在美国投人使用的溶剂吸收法中,三甘醇溶液使用就占到85%。
在我国,因考虑各类甘醇的产量及价格等因素,二甘醇和三甘醇均有采用;而我国海洋石油工业一般选用三甘醇溶液作为天然气脱水溶剂。
渤海友谊号FPSO (浮式生产储卸油装置)作为渤南油气田的生产处理中心,设置了原油处理系统、污水处理系统和天然气处理系统。
其中天然气处理系统包括天然气压缩系统和三甘醇脱水系统,分离出的天然气经增压和脱水后,通过单点和海底管线输往陆上终端,原油经脱水、稳定处理后去储油舱贮存,然后通过外输油轮外运。
友谊号FPSO 上三甘醇脱水及再生系统于2006年1月投入运行以来,相继出现了一些问题。
三甘醇脱水工艺认识及常见问题浅析
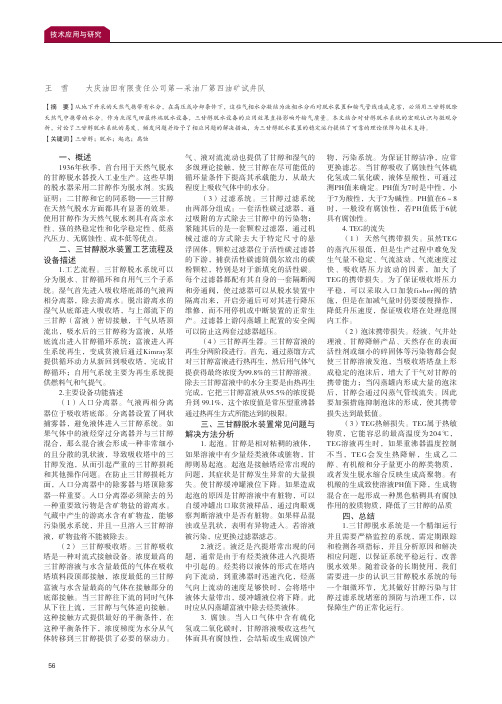
一、概述1936年秋季,首台用于天然气脱水的甘醇脱水器投入工业生产。
这些早期的脱水器采用二甘醇作为脱水剂。
实践证明:二甘醇和它的同系物——三甘醇在天然气脱水方面都具有显著的效果。
使用甘醇作为天然气脱水剂具有高亲水性、强的热稳定性和化学稳定性、低蒸汽压力、无腐蚀性、成本低等优点。
二、三甘醇脱水装置工艺流程及设备描述1.工艺流程。
三甘醇脱水系统可以分为脱水、甘醇循环和自用气三个子系统。
湿气首先进入吸收塔底部的气液两相分离器,除去游离水。
脱出游离水的湿气从底部进入吸收塔,与上部流下的三甘醇(富液)密切接触,干气从塔顶流出,吸水后的三甘醇称为富液,从塔底流出进入甘醇循环系统;富液进入再生系统再生,变成贫液后通过Kimray泵提供循环动力从新回到吸收塔,完成甘醇循环;自用气系统主要为再生系统提供燃料气和气提气。
2.主要设备功能描述(1)入口分离器。
气液两相分离器位于吸收塔底部。
分离器设置了网状捕雾器,避免液体进入三甘醇系统。
如果气体中的液烃穿过分离器并与三甘醇混合,那么混合液会形成一种非常细小的且分散的乳状液,导致吸收塔中的三甘醇发泡,从而引起严重的三甘醇损耗和其他操作问题。
在防止三甘醇损耗方面,入口分离器中的除雾器与塔顶除雾器一样重要。
入口分离器必须除去的另一种重要致污物是含矿物盐的游离水。
气藏中产生的游离水含有矿物盐,能够污染脱水系统,并且一旦溶入三甘醇溶液,矿物盐将不能被除去。
(2) 三甘醇吸收塔。
三甘醇吸收塔是一种对流式接触设备。
浓度最高的三甘醇溶液与水含量最低的气体在吸收塔填料段顶部接触,浓度最低的三甘醇富液与水含量最高的气体在接触部分的底部接触。
当三甘醇往下流的同时气体从下往上流,三甘醇与气体逆向接触。
这种接触方式提供最好的平衡条件,在这种平衡条件下,浓度梯度为水分从气体转移到三甘醇提供了必要的驱动力。
气、液对流流动也提供了甘醇和湿气的多级理论接触,使三甘醇在尽可能低的循环量条件下提高其承载能力,从最大程度上吸收气体中的水分。
三甘醇脱水再生废气回收利用的安全风险控制
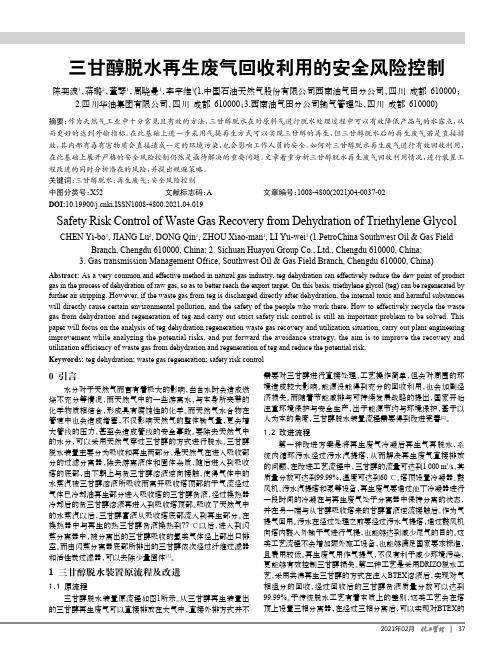
需要对三甘醇进行直接处理,工艺操作简单,但会对周围的环境造成较大影响。
能源没能得到充分的回收利用,也会加剧经济损失。
而随着节能减排与可持续发展战略的提出,国家开始注重环境保护与安全生产,出于能源节约与环境保护,基于以人为本的角度,三甘醇脱水装置流程需要得到改进完善[2]。
1.2 改进流程第一种改进方案是将再生废气冷凝后再生气再脱水,系统内循环污水经过污水汽提塔,从而解决再生废气直接排放的问题,在改进工艺流程中,三甘醇的流量可达到1 000 m 3/s ,其质量分数可达到99.99%,温度可达到60 ℃。
塔顶设置冷凝器、鼓风机、污水汽提塔和泵等设备,再生废气要通过他丁冷凝器进行一段时间的冷凝在与再生废气处于分离器中保持分离的状态,并在另一端与从甘醇吸收塔来的甘醇富液逆流接触后,作为气提气回用。
污水在经过处理之前要经过污水气提塔,通过鼓风机向塔内鼓入外输干气进行气提,也能够达到减少尾气的目的。
这类工艺流程不会增加额外施工设备,也能够满足国家要求标准,且费用较低。
再生废气用作气提气,不仅有利于减少环境污染,更能够有效控制三甘醇损失。
第二种工艺是采用DRIZO 脱水工艺,采用共沸再生三甘醇的方式在注入BTEX 溶液后,实现对气相组分的回收,经过回收后的三甘醇贫液质量分数可以达到99.99%。
于传统脱水工艺有着本质上的差别,这类工艺会在塔顶上设置三相分离器,在经过三相分离后,可以实现对BTEX 的0 引言水分对于天然气而言有着极大的影响,当含水时会造成燃烧不充分等情况,而天然气中的一些游离水,与本身所夹带的化学物质相结合,形成具有腐蚀性的化学。
而天然气水合物在管道中也会造成堵塞,不仅影响天然气的整体输气量,更会增大管线的压力,甚至会造成管线的安全事故。
要除去天然气中的水分,可以采用天然气穿过三甘醇的方式进行脱水。
三甘醇脱水装置主要分为吸收和再生两部分,是天然气在进入吸收部分的过滤分离器,除去游离液体和固体杂质,随后进入到吸收塔的底部,由下朝上与贫三甘醇溶液逆向接触,使得气体中的水蒸汽被三甘醇溶液所吸收而离开吸收塔顶部的干气流经过气体已冷却油再生部分进入吸收塔的三甘醇贫液,经过换热器冷却后的贫三甘醇溶液再进入到吸收塔顶部。
三甘醇再生系统活性炭滤芯费用优化措施
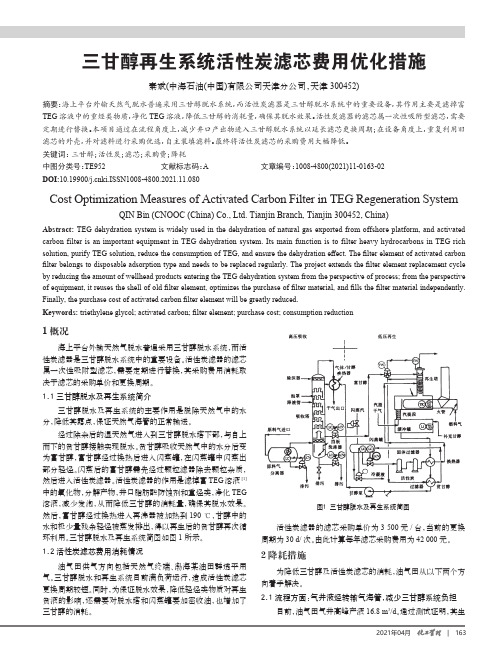
三甘醇再生系统活性炭滤芯费用优化措施秦斌(中海石油(中国)有限公司天津分公司,天津 300452)摘要:海上平台外输天然气脱水普遍采用三甘醇脱水系统,而活性炭滤器是三甘醇脱水系统中的重要设备,其作用主要是滤掉富TEG溶液中的重烃类物质,净化TEG溶液,降低三甘醇的消耗量,确保其脱水效果。
活性炭滤器的滤芯属一次性吸附型滤芯,需要定期进行替换。
本项目通过在流程角度上,减少井口产出物进入三甘醇脱水系统以延长滤芯更换周期;在设备角度上,重复利用旧滤芯的外壳,并对滤料进行采购优选,自主装填滤料。
最终将活性炭滤芯的采购费用大幅降低。
关键词:三甘醇;活性炭;滤芯;采购费;降耗中图分类号:TE952 文献标志码:A 文章编号:1008-4800(2021)11-0163-02DOI:10.19900/ki.ISSN1008-4800.2021.11.080Cost Optimization Measures of Activated Carbon Filter in TEG Regeneration System QIN Bin (CNOOC (China) Co., Ltd. Tianjin Branch, Tianjin 300452, China)Abstract: TEG dehydration system is widely used in the dehydration of natural gas exported from offshore platform, and activated carbon filter is an important equipment in TEG dehydration system. Its main function is to filter heavy hydrocarbons in TEG rich solution, purify TEG solution, reduce the consumption of TEG, and ensure the dehydration effect. The filter element of activated carbon filter belongs to disposable adsorption type and needs to be replaced regularly. The project extends the filter element replacement cycle by reducing the amount of wellhead products entering the TEG dehydration system from the perspective of process; from the perspective of equipment, it reuses the shell of old filter element, optimizes the purchase of filter material, and fills the filter material independently. Finally, the purchase cost of activated carbon filter element will be greatly reduced.Keywords: triethylene glycol; activated carbon; filter element; purchase cost; consumption reduction1概况海上平台外输天然气脱水普遍采用三甘醇脱水系统,而活性炭滤器是三甘醇脱水系统中的重要设备。
三甘醇再生系统温度自动控制介绍
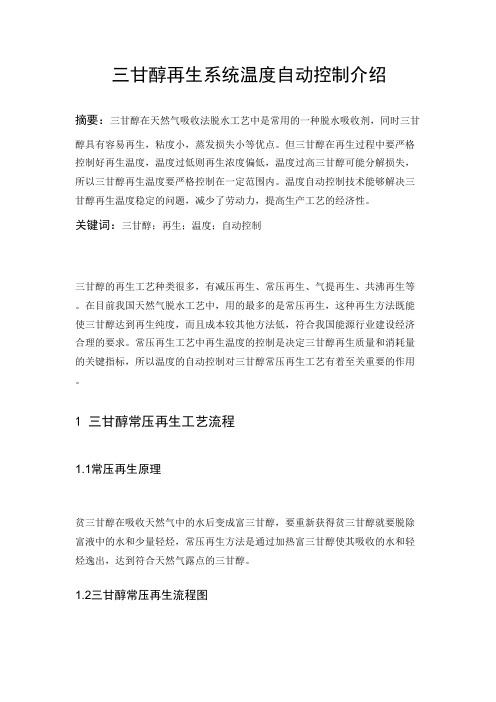
三甘醇再生系统温度自动控制介绍摘要:三甘醇在天然气吸收法脱水工艺中是常用的一种脱水吸收剂,同时三甘醇具有容易再生,粘度小,蒸发损失小等优点。
但三甘醇在再生过程中要严格控制好再生温度,温度过低则再生浓度偏低,温度过高三甘醇可能分解损失,所以三甘醇再生温度要严格控制在一定范围内。
温度自动控制技术能够解决三甘醇再生温度稳定的问题,减少了劳动力,提高生产工艺的经济性。
关键词:三甘醇;再生;温度;自动控制三甘醇的再生工艺种类很多,有减压再生、常压再生、气提再生、共沸再生等。
在目前我国天然气脱水工艺中,用的最多的是常压再生,这种再生方法既能使三甘醇达到再生纯度,而且成本较其他方法低,符合我国能源行业建设经济合理的要求。
常压再生工艺中再生温度的控制是决定三甘醇再生质量和消耗量的关键指标,所以温度的自动控制对三甘醇常压再生工艺有着至关重要的作用。
1 三甘醇常压再生工艺流程1.1常压再生原理贫三甘醇在吸收天然气中的水后变成富三甘醇,要重新获得贫三甘醇就要脱除富液中的水和少量轻烃,常压再生方法是通过加热富三甘醇使其吸收的水和轻烃逸出,达到符合天然气露点的三甘醇。
1.2三甘醇常压再生流程图三甘醇(TEG)富液从脱水吸收塔出来经过精馏柱换热后进入TEG闪蒸罐,初步分离出的富液依次进入机械过滤器、活性碳过滤器、机械过滤器进行过滤,除去其中的杂质,再进入缓冲罐与贫液换热后进入重沸器加热,蒸出其中的水分和轻烃,再生后的贫液进入缓冲罐与富液换热,出来后由TEG循环泵加压进入脱水吸收塔进行循环。
(汽提气可以在三甘醇提纯浓度不够使使用来提高提纯浓度,生产过程中由于经济型的考虑,一般不使用汽提气)2 重沸器温度控制参数2.1三甘醇再生温度范围确定随着再生温度的降低,贫液含水率呈增长趋势,此时水露点的变化规律与贫液含水率的变化规律基本一致,即贫液浓度增加,水露点降低;贫液浓度降低,水露点升高。
当TEG 再生温度为180 ℃时干气的水露点已经出现不合格的情况了,因此TEG 再生温度不宜低于180 ℃。
三甘醇再生废气处理工艺研究

三甘醇再生废气处理工艺研究荆国林;叶萍;吕广博【摘要】采出的天然气一般都为气态水所饱和,天然气中含有水会对设备和管道都有一定的损坏,也会降低天然气的热值.大庆天然气分公司北I-1深冷站使用三甘醇脱水工艺来处理天然气中含有的水分,三甘醇再生橇精馏柱排放出来的气体(以下简称三甘醇再生废气)中大部分为水蒸气,同时还含有甲烷、三甘醇和重烃,并带有异味.以前因排放出的气体没有实际利用价值而排放掉,不仅造成了周围环境的污染,而且严重危害企业员工的身心健康.在已有设备的基础上设计了一套分离吸附装置处理排出气,从而彻底解决排出废气处理的问题.经处理后的三甘醇废气中φ(三甘醇)由10.1%下降到0.3%,φ(重烃)由5.3%下降到0.02%,可以看出分离吸附装置具有良好的处理效果.【期刊名称】《化工科技》【年(卷),期】2015(023)005【总页数】3页(P24-26)【关键词】天然气脱水;三甘醇再生废气;分离吸附装置;去除率【作者】荆国林;叶萍;吕广博【作者单位】东北石油大学化学化工学院石油与天然气化工省高校重点实验室,黑龙江大庆163318;东北石油大学化学化工学院石油与天然气化工省高校重点实验室,黑龙江大庆163318;大庆油田有限责任公司天然气分公司,黑龙江大庆163000【正文语种】中文【中图分类】TE644大庆天然气分公司北I-1深冷站流出的天然气含有水分,其中的水分会结合成水合物堵塞并腐蚀管道和设备,降低运输能力[1-2]。
采用三甘醇脱水工艺处理天然气中含有的水分,但是排出的三甘醇再生废气带有异味,其中还含有少量的有害物质。
三甘醇再生废气直接排放到空气中,呼吸到人体内会对人体健康造成危害,也会对周围环境与周围设备有不同程度的损坏[3-5]。
因此解决三甘醇再生废气的排放问题迫在眉睫。
作者基于现有设备的基础上提出了一套处理三甘醇再生废气的技术,从根本上解决了三甘醇再生废气危害人体健康和污染环境的问题。
天然气脱水和三甘醇再生
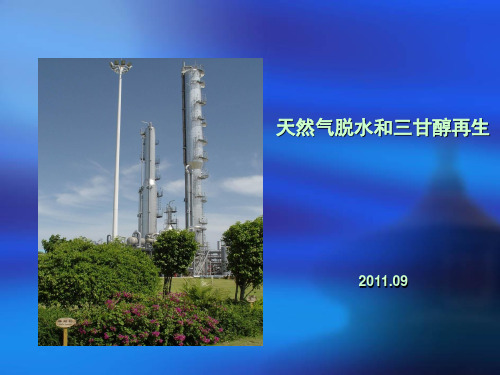
4.、必须满足一定的压力和温度条件.
防止水和物形成的方法: 1 、将天然气加热;2、采用液体(如三甘醇)或固 体(如分子筛)干燥剂将天然气脱水,使其露点降低;3、向气流中加入水合物抑 制剂。目前多采用热力学抑制剂(如甲醇、甘醇类),用来改变水溶液或水和物的 化学位,从而使水合物形成条件移向更低温度或更高压力范围。
5、贫气和富气
贫气:在20 ℃和101.325 Kpa的状态下,每立方米天然气中,丙烷以 上烃类按液态计小于100mL的天然气。 富气:在20 ℃和101.325 Kpa的状态下,每立方米天然气中,丙烷以 上烃类按液态计大于100mL的天然气。
6、天然气的热值
单位质量(体积)的天然气完全燃烧产生的热量,称为天然气的热 值。天然气的热值和天然气的组分有关,烃含量越高,热值越高。
天然气脱水工艺
天然气脱水工艺
1.3 干燥剂的选择
活性氧化铝 活性氧化铝是一种极性吸附剂,以部分水合的、多孔的无定形 AL2O3为主,并含有其他金属化合物(如氧化钠、三氧化二铁),比表 面即可达到250m2/g以上。例如,F-200活性氧化铝的组成为:氧化铝 94%、水5.5%、氧化钠0.3%、三氧化二铁0.02%。 由于活性氧化铝的湿容量大,故常用于水含量高的气体脱水。但 是,它在再生时能耗高,而且因其成碱性,可与无机酸发生化学反 应,故不宜处理酸性天然气。
W2 -- 图表1.2中H2S对应压力温度下的有效含水量
天然气脱水工艺
Y1 – CO2的摩尔百分数 Y2 – H2S的摩尔百分数 那么借助以上公式和图表, 我们就可以估算出如下生产条件下天然气中的含水量 : (以1550psig, 75℉, 10%CO2和0%H2S为工作条件) W = 90% X 22 + 10% X 150 + 0 X 2 = 35 lb / MMSCF 另外我们也可以估算出在下面条件下的天然气饱和水汽含量, 当时的工作条件是 : 1850 psig, 90 ℉ 和 10%CO2, 所以: W = 90% X 36 + 10% X 180 = 50 lb /MMSCF
生产应急处置-三甘醇再生系统闪蒸罐液位持续升高处理
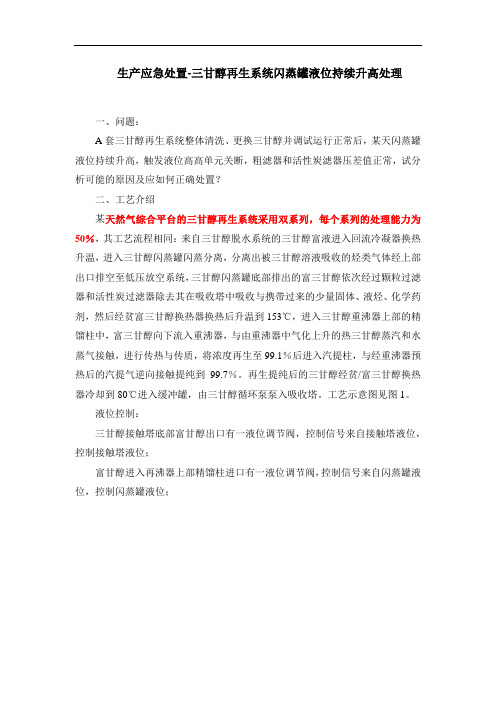
生产应急处置-三甘醇再生系统闪蒸罐液位持续升高处理一、问题:A套三甘醇再生系统整体清洗、更换三甘醇并调试运行正常后,某天闪蒸罐液位持续升高,触发液位高高单元关断,粗滤器和活性炭滤器压差值正常,试分析可能的原因及应如何正确处置?二、工艺介绍某天然气综合平台的三甘醇再生系统采用双系列,每个系列的处理能力为50%,其工艺流程相同:来自三甘醇脱水系统的三甘醇富液进入回流冷凝器换热升温,进入三甘醇闪蒸罐闪蒸分离,分离出被三甘醇溶液吸收的烃类气体经上部出口排空至低压放空系统,三甘醇闪蒸罐底部排出的富三甘醇依次经过颗粒过滤器和活性炭过滤器除去其在吸收塔中吸收与携带过来的少量固体、液烃、化学药剂,然后经贫富三甘醇换热器换热后升温到153℃,进入三甘醇重沸器上部的精馏柱中,富三甘醇向下流入重沸器,与由重沸器中气化上升的热三甘醇蒸汽和水蒸气接触,进行传热与传质,将浓度再生至99.1%后进入汽提柱,与经重沸器预热后的汽提气逆向接触提纯到99.7%。
再生提纯后的三甘醇经贫/富三甘醇换热器冷却到80℃进入缓冲罐,由三甘醇循环泵泵入吸收塔。
工艺示意图见图1。
液位控制:三甘醇接触塔底部富甘醇出口有一液位调节阀,控制信号来自接触塔液位,控制接触塔液位;富甘醇进入再沸器上部精馏柱进口有一液位调节阀,控制信号来自闪蒸罐液位,控制闪蒸罐液位;图1 三甘醇再生系统工艺示意图参考答案和评分标准(总分40分)可能原因:1)闪蒸罐液位计显示错误,但因液位持续升高,触发液位高高单元关断,综合液位高高开关被触发,说明闪蒸罐液位计实际较高,显示没有问题;(3分)2)接触塔液位变送器故障,液位调节阀开度变大,导致来液增多;(3分)3)接触塔液位控制阀阀芯冲蚀,全关时仍用流量流过,因为接触塔与闪蒸罐的压差较大,接近60公斤压差,可能存在冲蚀的可能性;4)粗滤器和活性炭滤器压差值显示正常,不存在滤器压差高堵塞原因;(3分)5)贫富甘醇换热器结垢,因A套三甘醇再生系统整体清洗、更换三甘醇并调试运行正常,内部较干净,时间较短,不存在结垢风险,可能性不大;(3分)6)闪蒸罐压力偏低,三甘醇从闪蒸罐到精馏柱压头不足,可能原因有闪蒸罐覆盖气管线外漏、闪蒸罐覆盖气进口SDV关闭、出口PCV设点漂移。
- 1、下载文档前请自行甄别文档内容的完整性,平台不提供额外的编辑、内容补充、找答案等附加服务。
- 2、"仅部分预览"的文档,不可在线预览部分如存在完整性等问题,可反馈申请退款(可完整预览的文档不适用该条件!)。
- 3、如文档侵犯您的权益,请联系客服反馈,我们会尽快为您处理(人工客服工作时间:9:00-18:30)。
TRIETHYLENE GLYCOL REGENERATION IN NATURAL GASDEHYDRATION PLANTS: A STUDY ON THE COLDFINGERPROCESSF. Gironi, M. Maschietti, V. Piemonte, Università degli Studi di Roma “LaSapienza”, D. Diba, S. Gallegati, S. Schiavo, Comart SpA, Ravenna, ItalyThis paper was presented at the Offshore Mediterranean Conference and Exhibition in Ravenna, Italy, March 28-30, 2007. It was selected for presentation by the OMC 2007 Programme Committee following review of information contained in the abstract submitted by the authors. The Paper as presented at OMC 2007 has not been reviewed by the Programme Committee. ABSTRACTNatural gas pipeline transportation requires very low water content in the gas stream in order to avoid condensation or hydrate formation. To reach this goal, when triethylene glycol (TEG) is used to dehydrate natural gas, after the absorption step TEG must be regenerated to levels substantially above 98.5-99.0 % by weight available from atmospheric distillation of glycol-water mixtures. In order to regenerate TEG to higher purity levels some of the methods used require a stripping gas, a solvent or to perform the distillation under vacuum. A simpler method to perform a further dehydration of TEG is the use of a water exhauster, known as Coldfinger, where the vapour in equilibrium with the liquid to be dehydrated is continuously condensed and removed. In this work, the Coldfinger apparatus was modelled and a study on the most relevant operating parameters was carried out. A process simulation of a natural gas dehydration plant, provided with a Coldfinger water exhauster for TEG regeneration, was performed on a case study. It was shown that the dehydration process with Coldfinger unit is capable of reaching current water content specifications in a simple and economic way.INTRODUCTIONNatural gas at the producing well contains significant quantities of water vapour. Typically, the gas is water-saturated at the condition of pressure and temperature of the well and a dehydration process is required. In fact, water content must be reduced in order to prevent liquid water condensation and hydrate formation in the pipeline transportation system. Nowadays, typical values of allowable water content in the gas transmission lines range from 70 to 120 mg/Nm3 [1].Among methods available for natural gas dehydration, absorption by means of triethylene glycol (TEG) is one of the most common. Water removal from the gas stream takes place by means of countercurrent contact between the gas, fed to the bottom of a contactor tower, and TEG, which is a liquid with a great affinity for water, fed at the top of it.The crucial part of the process is represented by TEG regeneration. If the water-rich TEG is distilled in a simple atmospheric column, TEG can not be regenerated to levels above 98.8-98.9 % by weight. This is caused by the reboiler operating temperature, which can not be fixed at temperature above 204 °C. In fact, this tempe rature must be regarded as an upper limit for TEG processing, because of thermal degradation at higher values [2,3].In the past these regeneration levels were sufficient because values of allowable water content in the lean gas were higher and regeneration was commonly performed in a simple atmospheric still column. On the other hand, in order to reach current water content specifications, it is necessary to regenerate TEG up to levels substantially above 99.0 % by weight.Fig 1: Water content in the dehydrated gas as a function of liquid to gas ratio in the absorption column. Case study process parameters: contactor theoretical stages 4, contactor pressure 50 bar, wet gas temperature 40 °C, TEG temperature at the inletport 100 °C.As an example referred to a typical natural gas dehydration case study, Fig. 1 shows water content in the lean gas as a function of the liquid to gas ratio in the absorption column. The curves, obtained by process simulation, refer to four different values of regenerated TEG mass fraction. This case study clearly shows that it is necessary to obtain TEG regeneration levels in the range 99.5-99.9 % by weight in order to dehydrate natural gas to current specifications. Fig. 1 also shows that for low regeneration levels (98.5-99.0 % by weight) a further increase in the liquid to feed ratio in the absorption column has no effect and can not allow to reach water content specifications. At low regeneration levels, also increasing the number of theoretical stages of the contactor does not allow to reach water content specifications.For these reasons, several alternative regeneration processes (vacuum distillation, stripping gas, azeotropic distillation, etc.) have been proposed in order to enhance TEG regeneration levels. If TEG-water distillation is performed under vacuum, TEG can be regenerated up to 99.9 % by weight, but high operating costs and plant control complexity are serious drawbacks. Another possibility is represented by using some of the dehydrated natural gas as a stripping gas in order to further dehydrate TEG. The contact with lean TEG exiting the still column can take place in the surge drum where TEG make-up is introduced or in an appropriate stripping column. Operating in this way lean TEG purity can be increased above 99.9 % by weight, but the consumption of some of the dehydrated gas represents an economic loss. Furthermore, the use of stripping gas causes a considerable increase of the gas send to flare, unless an expensive recovery system is arranged. Purity of lean TEG exiting the still column can also be enhanced by using a circulating solvent (such as heptane or octane) to perform an azeotropic distillation instead of the simple TEG-water distillation. In this way lean TEG concentration can be increased up to 99.99 % by weight but plant complexity and costs are increased because of the need of a three-phase separator at the top of the distillator, the needs of treatments for the oily water discharged from this separator and devices for the solvent circulation line (such as a circulation pump and a heater) [4].If the required purity of the lean TEG is in the range 99.0–99.9 % by weight, Coldfinger process [5,6] is a valuable alternative. This process is similar to the conventional dehydration process, except for the use of an apparatus, also called water exhauster, where the liquid stream exiting the reboiler of the still column is further dehydrated. Coldfinger process is advantageous because no stripping gas, solvent or further energy consumption are required and because of the simplicity of the water exhauster which can be easily installed as an addition to a conventional plant.In this work, a study on the performance of the Coldfinger regeneration process, in the context of natural gas dehydration, is carried out and a discussion on the influence of the most relevant process parameters is presented.THE COLDFINGER WATER EXHAUSTERIn Fig. 2 a schematic representation of the Coldfinger water exhauster is reported. The liquid stream exiting the reboiler of the still column is conveyed to the Coldfinger apparatus where a further dehydration takes place. The liquid flows horizontally through the lower portion of the apparatus, which is provided with some buffles to maintain the surface of the liquid agitated and turbulent in order to increase the vaporization rate. The liquid can be considered in equilibrium with its vapour, which occupies the remaining part of the vessel. In the upper part of the apparatus, an elongated horizontally disposed condenser (the “cold finger”) occupies a little part of the vapour space and is in contact with the vapour. The cold U tubes of the condenser cause a local condensation of the vapour in close proximity. Because of free convection and local condensation on the tubes, new vapour is drawn from the bulk to the proximity of the condenser, whereas the uncondensed and cooled vapour comes back and mixes again with the bulk. Droplets of condensed liquid gravitate to a collector shaped in a way to allow the liquid to flow and to be removed from the vessel. Removed liquid, which is a TEG-water mixture, can be refluxed to the still column or to its reboiler in order to be distilled again. Since the vapour is richer in water with respect to the liquid, as a result a further dehydration of the liquid flowing in the lower part of the apparatus is obtained. The pressure inside the vessel can be conveniently maintained at values nearly atmospheric by means of a gas regulation line, which can be fed by splitting minimal quantities of lean gas produced in the same dehydration unit.If the Coldfinger apparatus is fed by a liquid mixture about 98.8-98.9 % TEG by weight, which is the typical concentration of the liquid exiting the still column reboiler at 204 °C and 1.1 bar, TEG purity can be increased up to 99.5-99.9 % by weight operating at atmospheric pressure. Dehydration levels which is possible to obtain in the Coldfinger apparatus basically depend on the rate of heat removal by the condenser and on the rate of hot vapour rising to the proximity of the cold tubes.Fig 2: Schematic representation of the Coldfinger apparatus.These parameters depend on the extension of the cold surface of the condenser, the temperature of the refrigerating fluid and on the fluid dynamics of the two phases inside the apparatus.GAS DEHYDRATION PROCESS WITH COLDFINGER TEG REGENERATIONA typical flow scheme of the natural gas dehydration process, with TEG regeneration enhanced by the Coldfinger apparatus, is reported in Fig. 3.Wet gas is fed to the bottom of the absorption column (A), whereas regenerated TEG (13) is fed to the top of it. As a consequence of the contact between gas and TEG, a stream of dry gas is obtained from the top, whereas a stream of water-rich TEG (1) is withdrawn from the bottom of the column and is conveyed to the regeneration section. Since the absorption column operates at high pressure (typically 40-80 bar) and low temperature (typically 20-60 °C), whereas the regeneration section operates at press ure around 1 bar and high temperature (up to 204 °C), water-rich TEG is expande d and heated before entering the still column (S). In order to heat water-rich TEG, heat can be conveniently recovered from the regeneration section. In particular, water-rich TEG after expansion (2) can be pre-heated while removing heat at the Coldfinger tubes and then (3) at the condenser of the still column. Because of heating and reducing pressure, some flash gas is produced and separated from the liquid stream in a flash drum (D). After filtration (F) to remove impurities, water-rich TEG undergoes another pre-heating step in a heat-exchanger (H), recovering heat from lean regenerated TEG (11), and then is fed to the still column.Water-rich TEG is distilled in the still column. The reboiler (R) can be heated by exhaust gases of the combustion of a small fraction of natural gas produced in the unit and used as a fuel. Reboiler temperature must be limited at 204 °C to avoid TEG thermal degradation. In this condition, TEG mass fraction at the reboiler will be around 98.8-98.9 % by weight. In order to increase TEG mass fraction, this liquid stream is conveyed to the Coldfinger apparatus (C), which can be realized in a separate vessel or in a vessel integrated with the reboiler itself, as in the case of Fig. 3. Heat removal at the condenser placed in the upper part of the Coldfinger apparatus can be conveniently provided by water-rich TEG as explained above.Fig 3: Typical flow scheme of natural gas dehydration with Coldfinger regenerationprocess.As an alternative, if a greater amount of heat removal is required in order to reach higher regeneration levels, cooling water can be employed to give a lower temperature of the cold tubes. Condensed liquid on the “cold fingers” is reintroduced inside the column. Because of the small quantity of this liquid stream, its inlet point has negligible effect and so it can be conveniently reintroduced inside the reboiler. Regenerated TEG exiting the Coldfinger apparatus is cooled (H) as far as possible before being pumped (P) back to the absorption column.PROCESS SIMULATIONThe process represented in Fig. 3 was studied by means of the process simulator Hysys. Phase equilibria conditions were represented by means of a thermodynamic model based on the Peng-Robinson equation of state. Correct representation of phase equilibria conditions of the binary system TEG-water, especially at high temperature and for mixture composition close to water infinite dilution, proved to be an essential requirement for regeneration process design. A thermodynamic study on TEG-water system was carried out on published data in order to optimize equation parameters. Therefore, to represent binary TEG-water system, simulator data bank was not used. Details on thermodynamic modelling are provided elsewhere [7].Since commercial process simulators do not provide Coldfinger apparatus by default, an user-defined external routine (Coldfinger routine) was generated in order to include the water exhauster in the whole process scheme. At first, Coldfinger routine was tested separately by considering an inlet liquid at typical reboiler conditions (around 200 °C, 1.1 bar and TEG 98.9 % by weight) and varying the heat removal at the condenser, for three different liquid feed flow rates.Fig. 4 shows TEG mass fraction in the liquid stream exiting the bottom of the water exhauster as a function of the rate of heat removal. As it was expected, increasing heat removal leads to an increase of liquid dehydration. Furthermore, it can be noticed that the shape of the curves resembles the behaviour of condensation degree, as a function of heat removal, in an isobaric cooling of a vapour mixture with some uncondensable gas. In the first region of the curves, that is for small amounts of heat removal, the slope is quite low, which is to say dehydration is not very effective. Increasing the rate of heat removal a sudden change in the slope of the curves is found. In this region, condensation degree at the cold tubes increases considerably. As a result, a great amount of water is removed from the system, causing a significant liquid dehydration. At very high heat removal rates, the slope of the curves suddenly decreases again.Fig 4: Regenerated TEG mass fraction obtained by means of the Coldfinger water exhauster as a function of the heat removal. Inlet liquid conditions: 204 °C, 1.1 bar,TEG mass fraction 98.9 %This is because in this region an almost complete condensation of the vapour in the proximityof the cold tubes is obtained. Since the system contains also small quantities of gas, the uncondensed gas is strongly cooled. When it mixes back with the bulk, it starts to cool the whole system slowing down new vapourization from the liquid. As a consequence, further dehydration is strongly reduced.After testing the water exhauster alone, the Coldfinger routine was included in the whole process scheme of simulation. Thus, an analysis on the performance of the natural gas dehydration with Coldfinger regeneration process was carried out on a case study. A typical wet gas composition was selected, as reported in Table 1. In some cases, depending on wet gas temperature, a pre-dehydration was obtained by separation of a liquid phase before entering the absorption column. Other process parameters were fixed as follows:- Total inlet gas flow rate: 100000 kg/h;- Absorption column pressure: 50 bar;- Absorption column theoretical stages: 4- Still column theoretical stages: 3- Still column reflux ratio: 0.10The number of theoretical stages of the absorption column was not increased above the reported value because this parameter has a poor effect on gas dehydration, especially when compared with regenerated TEG purity, which is the key factor of the process. Similarly, the number of theoretical stages at the still column was kept at the reported low value because no further effect on TEG dehydration can be achieved by increasing this parameter. Still column reflux ratio was fixed at the minimal value which allowed to reduce TEG losses around zero (0.001 % by weight on the circulating glycol). As for the absorption column, TEG losses were less than 0.1 % by weight in all simulations.The process was studied for different wet gas temperatures (from 20 to 60 °C), varying the liquid to gas ratio (L/G) at the absorber column in the range 0.03-0.13, which is to say that circulating TEG was varied from 3000 to 13000 kg/h. Heat removal at the Coldfinger was realized exchanging with TEG from the absorption column, which is the more convenient solution. Continuous lines in Fig. 5 show the results obtained by these simulations. As it was expected, process performance is strongly influenced by the temperature of the wet gas entering the absorption column. In fact, the temperature profile of the absorption column depends primarily on the wet gas temperature, because of the low (L/G) ratio. Furthermore,at higher wet gas temperature, the ratio (L/G) has a greater influence on the dehydration level that is possible to obtain.Tab. 1: Natural (wet) gas composition selected for the case study.Total inlet gas flow rate: 100000 kg/h.Component Weight fractionMethane 0.3853Ethane 0.2408Propane 0.1948n-Butane 0.0687iso-Butane 0.0325C5 and superior 0.0592Water 0.0044Nitrogen 0.0122Carbon Dioxide 0.0021Fig 5: Water content in the lean gas as a function of the liquid to feed ratio in the absorption column referred to four different wet gas temperature. Continuous lines refer to Coldfinger regeneration process. Dashed lines refer to regeneration bymeans of stripping gas.In the case study under consideration, the use of the Coldfinger apparatus allows to reach typical water content specifications for wet gas temperature up to 50 °C. At lower gas temperatures, the process is more efficient for two reasons: favourable thermodynamic conditions at the absorption column and improvement of Coldfinger regeneration because of the lower temperature of water-rich TEG, which can provide a larger heat removal rate at the “cold fingers”. However, even at 60 °C is possible to str ongly reduce water content in the dehydrated gas stream, down to 200 mg/Nm3approximately. In this case, a further improvement can be obtained refrigerating the Coldfinger with cold water from an external circuit, in order to reach higher levels of regenerated TEG purity.Continuous lines in Fig. 6 report natural gas consumption for the dehydration process with Coldifnger regeneration enhancement. Reported values refer to two different wet gasC.temperatures: 20 and 60 °lines) for two gas temperatures.Gas is consumed at the burner of the still column reboiler in order to produce the vapour rising into the column. Values range from around 20 to 70 kg/h, depending on the circulating liquid flow rate. At 20 °C gas consumption is lower b ecause some water is separated before entering the absorption column, thus leading to a smaller boiling up at the reboiler.A classical dehydration process scheme, with TEG regeneration enhanced by using some of the dehydrated gas, was simulated in order to provide a comparison with the Coldfinger process. Process flow scheme is the same as Fig. 3, except for the absence of the Coldfinger, which is substituted by a stripping column fed by some gas splitted from dehydrated gas stream. The number of theoretical stages of the stripping column was fixed at 2, which is a typical value for this process. All the other process parameters were kept equal to the values reported above. In a preliminary set of simulations, stripping gas flow rate was varied until similar water content specifications, with respect to Coldfinger process, were obtained. This similarity was found operating the stripping column with a gas flow rate equal to 100 kg/h. The dependence of water content on liquid to gas ratio for this process is represented by the dashed lines shown in Fig. 5. Operating the stripping column at a constant gas flow rate, a minimum water content was found at high liquid to gas ratio. This is caused by two contrasting effects: increasing the circulating liquid improves the dehydration at the absorption column but regeneration becomes more difficult. This behaviour does not appear with Codfinger process because, when circulating TEG increases, heat removal rate at the “cold fingers” increases as well. A correct design of the Coldfinger can lead to a constant regeneration level while circulating liquid increases.Dashed lines in Fig. 6 show the consumption of dehydrated gas, with respect to the process with regeneration enhanced by stripping gas. Comparing the two processes, it can be seen that the Coldfinger process is capable of dehydrating natural gas consuming less dry gas, with respect to similar water content specifications. In particular, the gas consumption of the process with stripping gas regeneration resulted to be from 2.5 to 6 times greater than the Coldfinger process.CONCLUSIONSA study on the Coldfinger apparatus was carried out in order to underline its capability of further dehydrating TEG from atmospheric distillation. It was demonstrated that Coldfinger unit is capable of increasing TEG mass fraction up to 99.5 –99.8 % by weight, operating at atmospheric pressure. An analysis on the performance of the natural gas dehydration, including Coldfinger regeneration process, was carried out on a case study by means of the process simulator Hysys. It was demonstrated that current water specifications in the dehydrated gas can be reached without adding stripping gas, solvent or increasing energy consumption.REFERENCES[1] Huffmaster M.A., “Gas Dehydration Fundamentals”, Laurance Reid Gas Conditioning Conference, 2004[2] Steele W.V., Chirico R.D., Knipmeyer S.E., Nguyen A., “Vapor Pressure of Acetophenone, 1,2-Butanediol, 1,3-Butanediol, Diethylene Glycol Monopropyl Ether, 1,3-Dimethyladamantane, 2-Ethoxyethyl Acetate, Ethyl Octyl Sulfide, and Pentyl Acetate”, J.Chem.Eng.Data 41 (1996) 1255-1268[3] Rajeh A.O., Szirtes L., “Thermal Decomposition of Organic Derivatives of Crystalline Zirconium Phosphate – III. Thermal decomposition of diethylene glycol, benzyl alcohol and benzylamine intercalates of zirconium phosphate”, J. Thermal Analysis 37 (1991) 777-786[4] Shell, “Glycol Type Gas-Dehydration Systems”, Technical Manual, 2005[5] Reid L.S., “Apparatus for dehydrating organic liquids”, US Patent 3589984, 1971[6] Reid L.S., “Method of removing water from glycol solutions”, US Patent 4332643, 1978[7] Gironi F., Maschietti M., Piemonte V., "Modelling triethylene glycol-water system for natural gas dehydration", accepted for presentation at the Eight International Conference on Chemical and Process Engineering, Ischia, June 2007。