纳米氧化锌PPT幻灯片
几种典型纳米材料 ppt课件
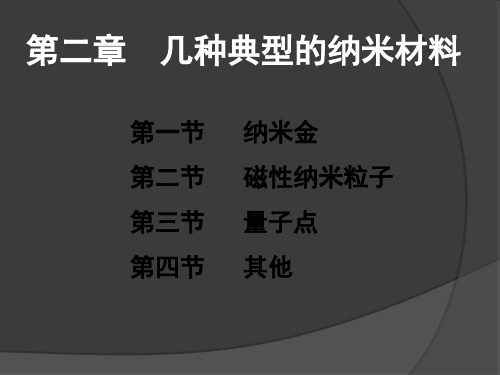
三、制备
(一)、制备方法 ——化学还原法
柠檬酸三钠法 柠檬酸三钠-鞣酸法 枸橼酸钠法 鞣酸-枸橼酸钠法 白磷法 抗坏血酸法 乙醇-超声波法 硼酸钠法
1、柠檬酸三钠法
1)取0、01%氯金酸(HAuCl4)水溶液100ml 加热至 沸,搅动下准确加入1%柠檬酸三 钠 (Na3C6H5O7.2H2O)水溶液 0.7ml,金黄色的氯金 酸水溶液在2分钟内变为紫红色,
改变鞣酸的加入量,制得的胶体颗粒大小不同。
3、枸橼酸三钠法
(1)10nm胶体金粒的制备:取0.01%HAuCl4水溶液100ml, 加入1%枸橼酸三钠水溶液3ml,加热煮沸30min,冷却至4℃, 溶液呈红色。
(2)15nm胶体金颗粒的制备:取0.01%HAuCl4水溶液100ml, 加入1%枸橼酸三钠水溶液2ml,加热煮沸15min~30min,直 至颜色变红。冷却后加入0.1Mol/L K2CO30.5ml,混匀即可。
胶金垫(Conjugate pad):
玻璃纤维、聚酯膜、纤维素滤纸、无纺布等多种材 质,多种规格,批间稳定。
结合垫的作用主要为:
- 吸附一定量的金标结合物颗粒; - 吸附并持续不断的将样品转移到NC膜上; - 保持金标结合物颗粒的稳定性; - 保证金标结合物颗粒定量完全释放等。
硝酸纤维素膜( Nitrocellulose):
硝酸纤维素膜与蛋白结合的原理 主要有两种假说:
1)首先两者靠静电作用力结合,然后靠H键和疏水作用来维持长 时间结合。 2)首先两者靠疏水作用结合,然后靠静电作用来维持长时间结合。 两条假说,都表明其结合过程分为两步,首先结合和后面长时间 结合。由于结合原理的不明确性,导致在这方面的工作非常依赖 实践经验。
4、枸橼酸三钠-鞣酸法
纳米氧化锌介绍与应用

纳米氧化锌介绍与应用纳米氧化锌(ZnO)粒径介于1-100 nm之间,是一种面向21世纪的新型高功能精细无机产品,表现出许多特殊的性质,如非迁移性、荧光性、压电性、吸收和散射紫外线能力等,利用其在光、电、磁、敏感等方面的奇妙性能,可制造气体传感器、荧光体、变阻器、紫外线遮蔽材料、图像记录材料、压电材料、压敏电阻、高效催化剂、磁性材料和塑料薄膜等。
概述中文名:纳米氧化锌英文名:Zinc oxide,nanometer 别名:纳米锌白;Zinc White nanometer CAS RN.:1314-13-2 分子式:ZnO 分子量:81.37形态纳米氧化锌是一种多功能性的新型无机材料,其颗粒大小约在1~100纳米。
由于晶粒的细微化,其表面电子结构和晶体结构发生变化,产生了宏观物体所不具有的表面效应、体积效应、量子尺寸效应和宏观隧道效应以及高透明度、高分散性等特点。
近年来发现它在催化、光学、磁学、力学等方面展现出许多特殊功能,使其在陶瓷、化工、电子、光学、生物、医药等许多领域有重要的应用价值,具有普通氧化锌所无法比较的特殊性和用途。
纳米氧化锌在纺织领域可用于紫外光遮蔽材料、抗菌剂、荧光材料、光催化材料等。
由于纳米氧化锌一系列的优异性和十分诱人的应用前景,因此研发纳米氧化锌已成为许多科技人员关注的焦点。
纳米氧化锌金属氧化物粉末如氧化锌、二氧化钛、二氧化硅、三氧化二铝及氧化镁等,将这些粉末制成纳米级时,由于微粒之尺寸与光波相当或更小时,由于尺寸效应导致使导带及价带的间隔增加,故光吸收显著增强。
各种粉末对光线的遮蔽及反射效率有不同的差异。
以氧化锌及二氧化钛比较时,波长小于350纳米(UVB)时,两者遮蔽效率相近,但是在350~400nm(UVA)时,氧化锌的遮蔽效率明显高于二氧化钛。
同时氧化锌(n=1.9)的折射率小于二氧化钛(n=2.6),对光的漫反射率较低,使得纤维透明度较高且利于纺织品染整。
纳米氧化锌还可用来制造远红外线反射纤维的材料,俗称远红外陶瓷粉。
纳米氧化锌综述

纳米氧化锌综述氧化锌(Zn0)晶体是纤锌矿结构.属六方晶系,为极性晶体。
Zn0晶体结构中,Zn原子按六方紧密堆积排列,每个Zn原子周围有4个氧原子,构成Zn--0配位四面体结构。
纳米氧化锌(Zn0)的性能和应用纳米氧化锌(Zn0)是一种白色粉末,是面向2l世纪的新型高功能精细无机产品,其粒径介于1~100nm。
由于颗粒尺寸的细微化,比表面积急剧增加,使得纳米氧化锌产生了其本体块状物料所不具有的表面效应、小尺寸效应和宏观量子隧道效应等,因而纳米氧化锌在磁、光、电、热、敏感等方面有一般氧化锌产品无法比拟的特殊性能。
1)制抗菌除臭、消炎、抗紫外线产品纳米氧化锌无毒、无味,对皮肤无刺激性,是皮肤的外用药物,能起消炎、防皱和保护等功效。
此外纳米氧化锌吸收紫外线的能力很强,对UVA(长波320~400nm)和UVB(中波280~320nm)均有良好的屏蔽作用。
可用于化妆品的防晒;也可以用于生产防臭、抗菌。
抗紫外线的纤维。
纳米氧化锌在阳光,尤其在紫外线照射下,在水和空气中,能分解出自有的带负电的电子,并同时留下带正电的空穴。
这种空血可以激活空气中的氧,使其变为活性氧,具有极强的化学活性,能与大多数有机物发生氧化反应,包括细菌体内的有机物,因而能杀死大多数的病毒。
纳米氧化锌的定量杀菌试验表明:在5min内,氧化锌的质量分数为1%试时,金黄色葡萄球菌的杀菌率为98.86%,大肠杆菌的杀菌率为99.93%。
2)用于氧化剂和光催化剂纳米氧化锌由于尺寸小、比表面积大、表面的键态与颗粒内部不同、表面原子配位不全等,导致表面的活性位臵增多,形成了凹凸不平的院原子台阶,增加了反应接触面。
因而纳米氧化锌的催化活性和选择性远远大于传统催化剂。
3)制备气体传感器及压电材料与SnO2、Fe2O3一起被称为气敏三大基体材料4)用于橡胶工业和涂料工业纳米氧化锌具有颗粒微小、比表面积大、分散性好、疏松多孔、流动性好等物理化学性质,因而,与橡胶的亲和性好,熔炼时易分散,胶料生热低、扯断变形小、弹性好,改善了材料工艺性能和物理性能。
纳米氧化锌
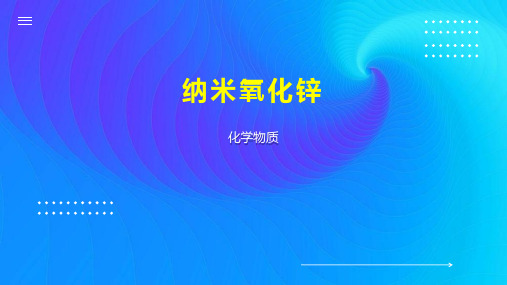
国家标准
中华人民共和国国家标准GB /T - 2004。 纳米氧化锌国家标准
产品前景
目前纳米氧化锌的制备技术已经取得了一些突破,在国内形成了几家产业化生产厂家。但是纳米氧化锌的表 面改性技术及应用技术尚未完全成熟,其应用领域的开拓受到了较大的限制,并制约了该产业的形成与发展。虽 然我们近年来在纳米氧化锌的应用方面取得了很大的进展,但与发达国家的应用水平以及纳米氧化锌的潜在应用 前景相比,还有许多工作要做。如何克服纳米氧化锌表面处理技术的瓶颈,加快其在各个领域的广泛应用,成为 诸多纳米氧化锌生产厂家所面临的亟待解决的问题。
减量使用
我们知道,氧化锌作为硫化体系必用的助剂,其填充量较高,一般为5份左右,由于氧化锌比重大,填充量大, 其对胶料密度的影响非常大。而动态使用的制品如轮胎等,重量越大,其生热、滚动阻力就愈大,对制品使用寿 命和能源消耗都不利,尤其是现代社会,人们对产品安全性和环保都提出了很高的要求。最近的国外名牌轮胎剖 析资料表明:其氧化锌用量远低于国内普通水平,一般约为1.5-2份左右。而国内以前由于材料的落后无法实现 这一点,现在大比表面纳米氧化锌的出现,可完全减量至这个水平,基本填补了这一空白。另外,减量使用对配 方成本的影响也较大,使通过减量使用降低成本成为现实。
1.平衡条件下反应动力学原理与强化的传热技术结合,迅速完成碱式碳酸锌的焙解。
2.通过工艺参数的调整,可以制备不同纯度、粒度及颜色的各种型号的纳米氧化锌产品。
3.本工艺可以利用多种含锌物料为原料,将其转化为高附加值产品。
4.典型绿色化工工艺,属于环境友好过程。
性能表征
纳米级氧化锌的突出特点在于产品粒子为纳米级,同时具有纳米材料和传统氧化锌的双重特性。与传统氧化 锌产品相比,其比表面积大、化学活性高,产品细度、化学纯度和粒子形状可以根据需要进行调整,并且具有光 化学效应和较好的遮蔽紫外线性能,其紫外线遮蔽率高达98%;同时,它还具有抗菌抑菌、祛味防霉等一系列独 特性能。
不同形貌的纳米氧化锌
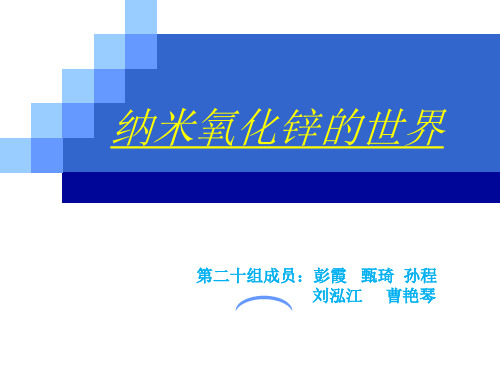
简单水热法制备棒状纳米氧化锌
表面活性剂 CTAB添 加量增加,制备产物对 次甲基蓝的光降解速 率降低 ——CTAB添加量增 加导致制备的ZnO棒 径增大,光降解表面变 小
棒径尺寸对纳米氧化锌光催化性能的影响
注:a 0.01mol/L CTAB b 0.1mol/L CTAB
——水热法
ZnO 纳米线
利用微波对系统加热 反应介质为有机相
可制备形貌特殊、且纯度较高的产品
微波加热法 溶剂热法
能够获得均匀粒子,反应时间也较水浴 加热大大缩短 能制备特殊形貌
水热法制备花状纳米氧化锌
配制 前驱体
0.6gZn(AcO)2 · 2H2O溶于3omL 蒸馏水中, 0.16g咪唑类离 子液体溶于 10mL蒸馏水中, 两者混合, 并搅拌10分钟
ቤተ መጻሕፍቲ ባይዱ
聚合物乳液进 一步修饰
带正电的多环 芳烃(2h)
水/去离子水洗
Pickering
棒状ZnO
——简单水热法
棒状纳米氧化锌的世界
简单水热法制备棒状纳米氧化锌
氧化锌纳米棒具有新奇的物化特性,纳米棒及其阵列具有优异的光电磁催化性 质,将对纳米元器件构筑和高级纳米功能材料的设计研究产生深远影响。
简单水热法
水热反应
后处理
将前驱体溶 液置于反应 釜中,180℃ 下加热24h
冷却至室温 ,将所得白 色产物分离 ,并用双蒸 水洗涤,于 60℃下干燥
——Maryam Movahedi, Elaheh Kowsari. Materials Letters, Volume 62, Issue 23, 31 August 2008
——SUN Ji-feng et al. Journal of Anhui Agri Sci ,2009, 37(27)
氧化锌基纳米材料的合成及其气敏性能-PPT课件

实验试剂: 硝酸锌 硝酸铝 柠檬酸 碳酸氢铵 乙二醇 硝酸铟 聚乙二醇 400 硝酸 氧化镧
2.2 材料的制备方法和表征
粉体材料的合成采用了室温固相法和 柠檬酸溶胶凝胶法,再将产物粉体经超 声波分散在乙醇中,然后在透射电子显 微镜或扫描电子显微镜下进行形貌观测, 并估测微粒粒径;通过射线衍射仪测定 材料的晶体结构,并进行物相分析。个 别样品在进行了热重差热分析。
图6-1 各样品的XRD 图谱
(a) ZnO (b) La2O3+ZnO (c) Al2O3+La2O3 + ZnO (d)Al2O3+ZnO
6.2 材料的表征(2)
图6-2a ZnO的TEM照片
图6-2b 样品Al2O3-La2O3-ZnO的TEM照片
图6-2为样品ZnO和Al2O3-La2O3-ZnO的TEM照片,按比 例估算粉体粒径分别为 50、20nm左右
6.4 元件对各种气体的选择性 由图可以看出: 在最佳工作温 度200℃下, 别的气体几乎 不会对Cl2的检 测产生干扰, 有望开发为高 选择性的氯气 敏感材料。
3.4 元件的响应恢复特性
t=290℃ 体积分数 =1×10-5 Tres = 7S Trec = 100S
图3-5 元件对Cl2的响应-恢复曲线
3.5 结论
本实验以Zn(NO3)2· 6H2O和NH4HCO3为原料通 过室温固相法制备了氧化锌纳米粉体,并且 借助XRD、SEM等测试手段对产品的粒径、 形貌进行了表征。研究表明该法制备的氧化 锌纳米粉体对Cl2表现出较好的灵敏度和选择 性,该制备方法省去了制备过程中的洗涤工 序,降低了制备过程中的设备要求,具有制 备简单,操作方便,且产率高等优点,有较 高的使用价值。
氧化锌znoppt课件
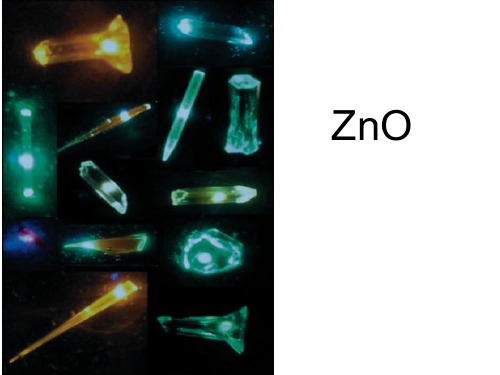
(a) 自由激子复合发光
自由激子(FE)可以在晶体中运动,传播能量和动量, 但不传播电荷。对于直接带结构半导体,自由激子发 光一般是来自n=1激子能级的跃迁,其发射能量为
exEg R*
其中R*为激子等效里德堡常数。对间接带结构半导体, FE激子发光还需要声子的参与,激子的发光能量为
exEgR*NpE
.
ZnO中的可见发光
• ZnO的可见发光是较宽的发光谱带,包括蓝 光、绿光、黄光、红光等波段。
• 通常认为ZnO的可见发光是与ZnO中的各种 本征缺陷,如间隙(Zni、Oi)、空位(Vo、 VZn)、反位(OZn、ZnO)等有关,或者是由 掺杂如Cu、Mn、Er等引起。
.
ZnO材料的优势
光电 热电 压电 铁电 铁磁
ZnO的光致发光性质
• 半导体材料的光学性质主要包括本征和非本征光 学过程。本征光学过程主要指带间的辐射和吸收 跃迁以及激子的跃迁。
• 非本征光学过程指的是由杂质和缺陷态所产生的 跃迁。通常可以通过发射光谱、吸收(透射)光谱 和激子光谱表征这些跃迁过程。
• 通常室温下ZnO的光致发光谱中包含两个波段, 一个是紫外发光峰,一个是可见发光峰。研究者 一般将紫外发光峰归结于自由激子发射及其声子 伴线。可见发光峰一般观点是来自于ZnO中的某 种本征缺陷如氧空位、氧反位等,或由外来杂质 引起的。
ZnO
.
ZnO的基本性质
• ZnO的分子量为81.39 • 密度为5.606g/cm3 • 无毒、无臭、无味、无砂性 • 属于两性氧化物。ZnO能溶于酸、碱以及氨水、
氯化钱等溶液,不溶于水、醇(如乙醇)和苯等有 机溶剂 • 熔点为1975℃,加热至1800℃升华但是不分解
.
ZnO的特性
纳米氧化锌材料
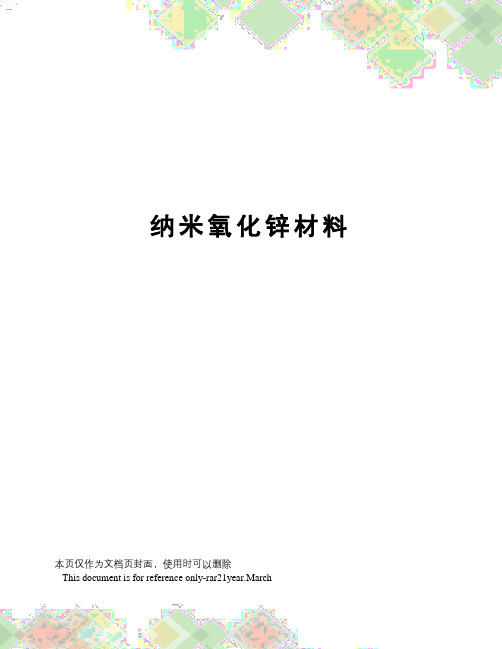
纳米氧化锌材料本页仅作为文档页封面,使用时可以删除This document is for reference only-rar21year.March纳米氧化锌材料研究现状[摘要]总之,纳米ZnO作为一种新型无机功能材料,从它的许多独特的用途可发现其在日常生活和科研领域具有广阔的市场和诱人的应用前景。
随着研究的不断深入与问题的解决,将有更多的优异性能将会被发现。
同时更为廉价的工业化生产方法也将会成为现实,纳米ZnO材料将凭借其独特的性能进入我们的日常生活。
随着科技的发展,相信纳米ZnO材料的性能及应用将会得到更大的提高和普及,并在新能源、环保、信息科学技术、生物医学、安全、国防等领域发挥重要的作用。
[关键词]纳米ZnO; 表面效应; 溶胶-凝胶法;纳米复合材料一、纳米氧化锌体的制备目前,制备纳米氧化锌的方法很多,归纳起来有属于液相法的沉淀法、溶胶-凝胶法、水热法、溶剂热法等,也有属于气相法的化学气相反应法等,而固相法在纳米氧化锌的制备领域则较少见。
a、沉淀法沉淀法是指使用某些沉淀剂如OH-、CO32-、C2O42-等,或在一定的温度下使溶液发生水解反应,从而析出产物,洗涤后得到产品[2]。
沉淀法一般有分为均匀沉淀法、络合沉淀法、共沉淀法等。
均匀沉淀法工艺成本低、工艺简单,为研究纳米氧化锌结构与性能及应用之间的关系提供了方便。
曾宪华[3]等人以常见且廉价的六水硝酸锌和氢氧化钠为以甲醇溶液作为溶剂在常温常压条件下,用均匀沉淀法直接制备了平均粒径为11 nm的纳米氧化锌粉体。
以下是他们的用共沉淀法制备的纳米ZnO 的扫描电子显微镜(SEM)照片。
络合沉淀法,制备的纳米Zn0不团聚,分散性好,粒径均匀。
李冬梅[4]等人采用络合沉淀法制备了粉体平均粒径52 nm,分散性好的纳米氧化锌粉体,并对产品结构性能进行了表征。
所得ZnO粉体平均粒径48 nm.分散性好,收率高。
共沉淀法是将含两种或两种以上的阳离子加入到沉淀剂中,使所有的离子同时完全沉淀。
《ZnO光电子学应用》PPT课件
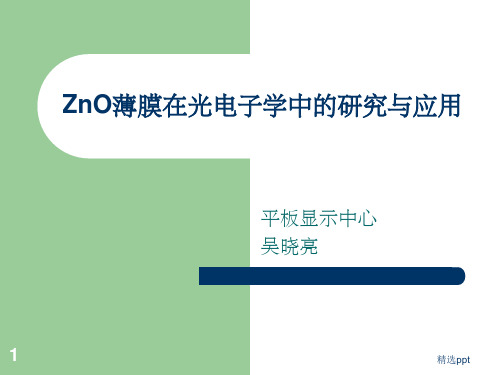
化合物
ZnO
Байду номын сангаас
晶体结构
纤锌矿
晶格常数 A
a=3.249, c=5.206
禁带宽度 eV
3.2
MO(M=Mg,Be,Cd)混溶实现 MgO 岩盐 4.22
7.9
ZnMO。
– ZnO与锌硫族化合物
CdO 岩盐
4.69
2.7
ZnX(X=S,Se)混溶实现 ZnOX。
三元合金一般有两种晶体结
4
Zn-4s
O-2p
5.0 51 04T2 E(T g)E(g T0)
900 T
精选ppt
ZnO的缺陷
错位与晶界
– ZnO薄膜中存在原生层错和扩展位错、ZnO薄膜中退火诱生 的层错及晶界
本征点缺陷
– 氧空位、锌空位(绿光)、氧间隙、锌间隙、反位缺陷 – 其中.氧空位和锌间隙之类的“缺氧”环境对ZnO的P型掺杂
7
段近似。
精选ppt
ZnO薄膜的制备技术
ZnO-TCO薄膜的制备技术
关于成膜技术, PLD法与磁控溅射法是 制得高质量的ZnO的较 好方法但是设备复杂 效率低,成本高, MOCVD成膜质量较好但 成本也较高,喷雾热 分解法、Sol-gel和电 化学沉积法都可以实 现低成本大面积成膜 但是成膜质量一般工 艺有待改进。
仪表窗口
防雾防霜玻璃:汽车,飞机挡风玻璃,相机,滑雪眼镜
面发热膜
取暖用嵌板散热器
烹调用加热器
光学 方面
红外反射膜 选择投射莫
10
的应 用 气敏元件
节能红外反射器
建筑物装饰玻璃
电炉、干燥箱观察窗
太阳光聚热器
精选ppt
氧化锌纳米结构
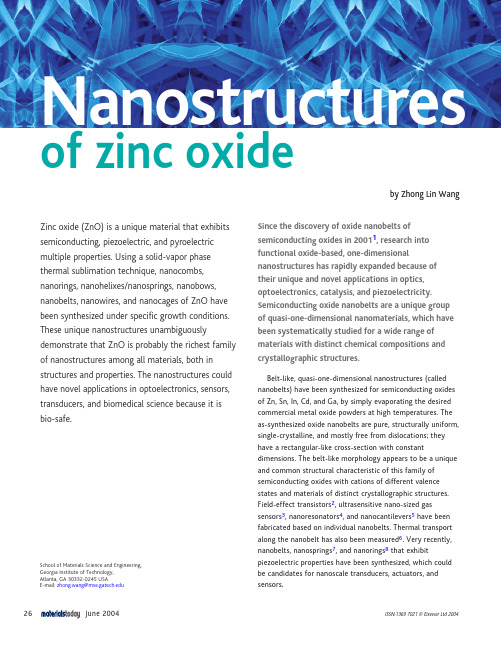
Since the discovery of oxide nanobelts of semiconducting oxides in 20011, research into functional oxide-based, one-dimensional nanostructures has rapidly expanded because of their unique and novel applications in optics,optoelectronics, catalysis, and piezoelectricity.Semiconducting oxide nanobelts are a unique group of quasi-one-dimensional nanomaterials, which have been systematically studied for a wide range of materials with distinct chemical compositions and crystallographic structures.Belt-like, quasi-one-dimensional nanostructures (called nanobelts) have been synthesized for semiconducting oxides of Zn, Sn, In, Cd, and Ga, by simply evaporating the desired commercial metal oxide powders at high temperatures. The as-synthesized oxide nanobelts are pure, structurally uniform,single-crystalline, and mostly free from dislocations; they have a rectangular-like cross-section with constantdimensions. The belt-like morphology appears to be a unique and common structural characteristic of this family of semiconducting oxides with cations of different valence states and materials of distinct crystallographic structures.Field-effect transistors 2, ultrasensitive nano-sized gassensors 3, nanoresonators 4, and nanocantilevers 5have been fabricated based on individual nanobelts. Thermal transport along the nanobelt has also been measured 6. Very recently,nanobelts, nanosprings 7, and nanorings 8that exhibitpiezoelectric properties have been synthesized, which could be candidates for nanoscale transducers, actuators, and sensors.by Zhong Lin WangNanostructuresof zinc oxideSchool of Materials Science and Engineering, Georgia Institute of Technology, Atlanta, GA 30332-0245 USAE-mail: zhong.wang@June 200426ISSN:1369 7021 © Elsevier Ltd 2004Zinc oxide (ZnO) is a unique material that exhibits semiconducting, piezoelectric, and pyroelectric multiple properties. Using a solid-vapor phase thermal sublimation technique, nanocombs,nanorings, nanohelixes/nanosprings, nanobows,nanobelts, nanowires, and nanocages of ZnO have been synthesized under specific growth conditions.These unique nanostructures unambiguouslydemonstrate that ZnO is probably the richest family of nanostructures among all materials, both in structures and properties. The nanostructures could have novel applications in optoelectronics, sensors,transducers, and biomedical science because it is bio-safe.REVIEW FEATUREAmong the functional oxides with perovskite, rutile, CaF 2,spinel, and wurtzite structures 9, ZnO is unique because it exhibits dual semiconducting and piezoelectric properties.ZnO is a material that has diverse structures, whose configurations are much richer than any knownnanomaterials including carbon nanotubes. Using a solid-state thermal sublimation process and controlling the growth kinetics, local growth temperature, and the chemical composition of the source materials, a wide range ofnanostructures of ZnO have been synthesized (Fig. 1). This review focuses on the formation of nanohelixes, nanobows,nanopropellers, nanowires, and nanocages of ZnO.Nanohelixes/nanosprings and seamless nanoringsThe wurtzite structure family has a few important members,such as ZnO, GaN, AlN, ZnS, and CdSe, which are importantmaterials for applications in optoelectronics, lasing, and piezoelectricity. The two important characteristics of the wurtzite structure are the noncentral symmetry and polar surfaces. The structure of ZnO, for example, can be described as a number of alternating planes composed of tetrahedrally coordinated O 2-and Zn 2+ions, stacked alternately along the c -axis (Fig. 2a ). The oppositely charged ions produce positively charged (0001)-Zn and negatively charged(0001)-O polar surfaces, resulting in a normal dipole moment and spontaneous polarization along the c -axis, as well as a divergence in surface energy.By adjusting the raw materials with the introduction of impurities, such as In, we have synthesized a nanoring structure of ZnO (Fig. 2)8. High-magnification scanning electron microscopy (SEM) images clearly show the perfect circular shape of the complete ring, with uniform shape andflat surfaces. Transmission electron microscopy (TEM) imagesFig. 1 A collection of nanostructures of ZnO synthesized under controlled conditions by thermal evaporation of solid powders. Most of the structures presented can be produced with 100%purity.June 200427indicate that the nanoring is a single-crystal entity with a circular shape. The single-crystal structure referred to here means a complete nanoring made of a single-crystalline ribbon bent evenly at the curvature of the nanoring. Thenanoring is the result of coaxial, uniradius, epitaxial coiling of a nanobelt.The growth of nanoring structures can be understood by considering the polar surfaces of the ZnO nanobelt. The polar nanobelt, which is the building block of the nanoring, growsalong [1010], with side surfaces ±(1210) and top/bottom surfaces ±(0001), and has a typical width of ~15 nm and thickness of ~10 nm. The nanobelt has polar charges on its top and bottom surfaces (Fig. 2b ). If the surface charges are uncompensated during growth, the nanobelt may tend to fold itself, as it lengthens, to minimize the area of the polar surface. One possible way is to interface the positively charged (0001)-Zn plane (top surface) with the negatively charged (0001)-O plane (bottom surface), resulting in neutralization of the local polar charges and reduction of the surface area, thus forming a loop with an overlapped end (Fig. 2b ). The radius of the loop may be determined by the initial folding of the nanobelt during early growth, but the size of the loop cannot be too small to reduce the elastic deformation energy. The total energy involved in the process comes from the polar charges, surface area, and elasticdeformation. The long-range electrostatic interaction is likely to be the initial driving force for the folding of the nanobelt to form the first loop for subsequent growth. As the growth continues, the nanobelt may be naturally attracted onto the rim of the nanoring because of electrostatic interactions and may extend parallel to the rim of the nanoring to neutralize the local polar charge and reduce the surface area. This results in the formation of a self-coiled, coaxial, uniradius,multilooped nanoring structure (Fig. 2c ). The self-assembly is spontaneous, which means that the self-coiling along the rim proceeds as the nanobelt grows. The reduced surface areaREVIEW FEATUREJune 200428Fig. 2 Seamless single-crystal nanorings of ZnO. (a) Structure model of ZnO, showing the ±(0001) polar surfaces. (b-e) Proposed growth process and corresponding experimental results showing the initiation and formation of the single-crystal nanoring via the self-coiling of a polar nanobelt. The nanoring is initiated by folding a nanobelt into a loop with overlapped ends as a result of long-range electrostatic interactions among the polar charges; the short-range chemical bonding stabilizes the coiled ring structure; and the spontaneous self-coiling of the nanobelt is driven by minimization of the energy contributed by polar charges, surface area, and elastic deformation. (f) SEM images of the as-synthesized, single-crystal ZnO nanoring. (g) The ‘slinky’ growth model of the nanoring. (h) The charge model of an α-helix protein, in analogy to the charge model of the nanobelt during the self-coiling process.REVIEWFEATUREFig. 3 (a) Model of a polar nanobelt. Polar-surface-induced formation of (b) nanorings, (c) nanospirals, and (d) nanohelixes of ZnO and their formation processes.June 200429and the formation of chemical bonds (short-range forces)between the loops stabilize the coiled structure. The width of the nanoring increases as more loops wind along thenanoring axis, and all remain in the same crystal orientation (Fig. 2d ). Since the growth is carried out in a temperature region of 200-400°C, ‘epitaxial sintering’ of the adjacent loops forms a single-crystal cylindrical nanoring structure,and the loops of the nanobelt are joined by chemical bonds into a single entity (Fig. 2e ). A uniradius, perfectly aligned coiling is energetically favorable because of the complete neutralization of the local polar charges inside the nanoring (Fig. 2f ) and the reduced surface area. This is the ‘slinky’growth model of the nanoring shown in Fig. 2g . The charge model of the nanoring is analogous to the α-helix protein molecule (Fig. 2h ).We have recently synthesized ZnO nanobelts that are dominated by the (0001) polar surface 7. The nanobelt grows along [2110] (the a -axis), with its top/bottom surfaces thinness (5-20 nm) and large aspect ratio (~1:4), theflexibility and toughness of the nanobelts is extremely high. A polar-surface-dominated nanobelt can be approximated to be a capacitor with two parallel charged plates (Fig. 3a ). The polar nanobelt tends to roll over into an enclosed ring to reduce the electrostatic energy (Fig. 3b ). A spiral shape can also reduce the electrostatic energy (Fig. 3c ). The formation of the nanorings and nanohelixes can be understood from the nature of the polar surfaces. If the surface charges are uncompensated during the growth, the spontaneouspolarization induces electrostatic energy as a result of the dipole moment. But rolling up to form a circular ring would minimize or neutralize the overall dipole moment, reducing the electrostatic energy. On the other hand, bending the nanobelt produces elastic energy. The stable shape of the nanobelt is determined by the minimization of the total energy contributed by spontaneous polarization and elasticity.If the nanobelt is rolled uniradially loop-by-loop, the repulsive force between the charged surfaces stretches the nanohelix, while the elastic deformation force pulls the loops together; the balance between the two forms thenanohelix/nanospring shown in Fig. 3d . The nanohelix has a uniform shape with a radius of ~500-800 nm and evenly distributed pitches. Each is made of a uniformly deformed single-crystal ZnO nanobelt.The striking new feature of the nanorings and nanohelixes of single-crystalline ZnO nanobelts reported here is that they are spontaneous-polarization-induced structures, the result ofa 90° rotation in polarization. These are ideal objects for understanding piezoelectricity and polarization-induced phenomena at the nanoscale. The piezoelectric nanobelt structures could also be used as nanoscale sensors, transducers, or resonators.Aligned nanopropellersModifying the composition of the source materials can drastically change the morphology of the grown oxide nanostructure. We used a mixture of ZnO and SnO2powders in a weight ratio of 1:1 as the source material to grow a complex ZnO nanostructure10,11. Fig. 4a is an SEM image of the as-synthesized products showing a uniform feature consisting of sets of central axial nanowires, surrounded by radially oriented ‘tadpole-like’ nanostructures. The morphology of the string appears like a ‘liana’, while the axial nanowire is like ‘rattan’, which has a uniform cross-section with dimensions in the range of a few tens of nanometers. The tadpole-like branches have spherical balls at the tips (Fig. 4a), and the branches display a ribbon shape. The ribbon branches have a fairly uniform thickness, and their surfaces are rough with steps. Secondary growth on the ‘propeller’surface leads to aligned nanowires (Fig. 4b).It is known that SnO2can decompose into Sn and O2at high temperature, thus the growth of the nanowire-nanoribbon junction arrays is the result of the vapor-liquid-solid (VLS) growth process, in which Sn catalyst particles are responsible for initiating and leading the growth of ZnO nanowires and nanoribbons. The growth of the novel structures presented here can be separated into two stages. The first stage is fast growth of the ZnO axial nanowire along [0001] (Fig. 4c). The growth rate is so high that a slow increase in the size of the Sn droplet has little influence on the diameter of the nanowire, thus the axial nanowire has a fairly uniform shape along the growth direction. The second stage is the nucleation and epitaxial growth of nanoribbons as a result of the arrival of tiny Sn droplets onto the ZnO nanowire surface (Fig. 4d). This stage is much slower than the first one because the lengths of the nanoribbons are uniform and much shorter than that of the nanowire. Since Sn is in a liquid state at the growth temperature, it tends to adsorb the newly arriving Sn species and grows into a larger size particle (i.e. coalesces). Therefore, the width of the nanoribbon increases as the size of the Sn particle at the tip becomes larger, resulting in the formation of the tadpole-like structure observed in the TEM (Fig. 4e). The Sn liquid droplets deposited onto the ZnO nanowire lead to the simultaneous growth of ZnO nanoribbons along six equivalent growth directions: ±[1010], ±[0110], and ±[1100]. Secondary growth along [0001] results in the growth of aligned nanowires on the surfaces of the propellers (Fig. 4f).Patterned growth of aligned nanowires The growth of patterned and aligned one-dimensional nanostructures is important for applications in sensing2,3, optoelectronics, and field emission12,13. Aligned growth of ZnO nanorods has been successfully achieved on a solid substrate via the VLS process with the use of Au14,15andSn16as catalysts, which initiate and guide the growth. The epitaxial orientation relationship between the nanorods and the substrate leads to aligned growth. Other techniques that do not use catalysts, such as metalorganic vapor-phase epitaxial growth17, template-assisted growth12, and electrical field alignment18have also been employed for the growth of vertically aligned ZnO nanorods. Huang et al.have demonstrated a technique for growing periodically arranged carbon nanotubes using a catalyst pattern produced from aREVIEW FEATUREJune 200430Fig. 4 Nanopropeller arrays of ZnO. (a) SEM image of Sn-catalyzed growth of aligned nanopropellers based on the six equivalent crystallographic directions. (b) Secondary growth of nanowires on the surface of the nanopropellers. (c-f) Growth process of the nanopropellers.REVIEW FEATUREmask and self-assembled submicron spheres 19. We have combined this self-assembly-based mask technique with the surface epitaxial approach to grow large-area hexagonal arrays of aligned ZnO nanorods 20.The synthesis process involves three main steps. The hexagonally patterned ZnO nanorod arrays are grown on a single-crystal Al 2O 3substrate on which patterned Au catalyst particles have been dispersed. First, a two-dimensional, large-area, self-assembled and ordered monolayer of submicron polystyrene spheres is introduced onto the single-crystal Al 2O 3substrate (Fig. 5a ). Second, a thin layer of Au particles is deposited onto the self-assembled monolayer; the spheres are then etched away, leaving a patterned Au catalyst array (Fig. 5b ). Finally, nanowires are grown on the substrate using the VLS process (Fig. 5c ). The spatial distribution of the catalyst particles determines the pattern of the nanowires.This step can be achieved using a variety of masktechnologies for producing complex configurations. Bychoosing the optimum match between the substrate lattice and the nanowires, the epitaxial orientation relationshipbetween the nanowire and the substrate results in the aligned growth of nanowires normal to the substrate. The distribution of the catalyst particles defines the location of the nanowires, and the epitaxial growth on the substrate results in the vertical alignment.Mesoporous single-crystal nanowiresPorous materials have a wide variety of applications in bioengineering, catalysis, environmental engineering, and sensor systems because of their high surface-to-volume ratio.Normally, most of these mesoporous structures arecomposed of amorphous materials and porosity is achieved by solvent-based organic or inorganic reactions. There are few reports of mesoporous structures based on crystalline material.We have reported a novel wurtzite ZnO nanowire structure that is a single crystal but is composed ofmesoporous walls/volumes 21. The synthesis is based on a modified solid-vapor process. Fig. 6a shows an SEM image of the as-synthesized ZnO nanowires grown on a Si substrate coated with a thin layer of Sn catalyst. The typical length ofthe nanowires varies from 100 µm to 1 mm and the diameterFig. 5 Growth of patterned and aligned ZnO nanowires. (a) Self-assembled monolayer of polystyrene spheres that serves as a mask. (b) Hexagonally patterned Au catalyst on the substrate. (c) Aligned ZnO nanowires grown on a single-crystal alumina substrate in a honeycomb pattern defined by the catalyst mask.June 200431Fig. 6 Mesoporous, single-crystal ZnO nanowires. (a) SEM image of high-porosity ZnO nanowires grown on an Sn-coated Si substrate. (b) High-magnification SEM images showing the morphology of a single nanowire. (c) Low-magnification TEM image of a porous ZnO nanowire and corresponding electron diffraction pattern, showing that the ZnO porous wire is covered by a thin layer of Zn 2SiO 4.is in the range of 50-500 nm. The porous structure of the ZnO nanowires is apparent (Fig. 6b ). A corresponding electron diffraction pattern from the nanowire presents two sets ofZn 2with a standard deviation of ±1.5 nm, indicating a very good size uniformity.To examine size-induced quantum effects in the ultrathin ZnO nanobelts, photoluminescence (PL) measurements were performed at room temperature using an Xe lamp with an excitation wavelength of 330 nm (Fig. 7b ). In comparison with the PL measurements from nanobelts with an average width of ~200 nm, the 6 nm nanobelts show a 14 nm shift in the emission peak, which possibly indicates quantumconfinement arising from the reduced size of the nanobelts.Polyhedral cagesCages of ZnO have also been synthesized at a high yield and purity 23. The mesoporous-structured polyhedral drum and spherical cages and shells are formed by textured self-assembly of ZnO nanocrystals, which are made by a novel self-assembly process during epitaxial surface oxidation (Fig. 8) The cages and shells exhibit unique geometricalnanostructures has been grown. It canREVIEW FEATUREJune 200432Fig. 7 Ultra-narrow ZnO nanobelts. (a) TEM image of a ZnO nanobelt grown using a Sn thin-film catalyst. (b) PL spectra of the wide (W = 200 nm) and narrow nanobelts (W = 6 nm), showing the blue shift in the emission peak as a result of size effects.REVIEW FEATUREbe predicted that ZnO is probably the richest family of nanostructures among all one-dimensional nanostructures,including carbon nanotubes.ZnO has three key advantages. First, it is semiconductor,with a direct wide band gap of 3.37 eV and a large excitation binding energy (60 meV). It is an important functional oxide,exhibiting near-ultraviolet emission and transparentconductivity. Secondly, because of its noncentral symmetry,ZnO is piezoelectric, which is a key property in building electromechanical coupled sensors and transducers. Finally,ZnO is bio-safe and biocompatible, and can be used forbiomedical applications without coating. With these three unique characteristics, ZnO could be one of the mostimportant nanomaterials in future research and applications.The diversity of nanostructures presented here for ZnOshould open up many fields of research in nanotechnology. MTAcknowledgmentsThanks to Y. Ding, P. X. Gao, W. L. Hughes, X. Y. Kong, C. Ma, D. Moore, Z. W. Pan,C. J. Summers, X.D. Wang, and Y. Zhang for helpful discussion and their contributions to the work reviewed in this article. We acknowledge generous support by the Defense Advanced Projects Research Agency, National Science Foundation, and NASA.June 200433REFERENCES1.Pan, Z. W., et al ., Science (2001) 291, 19472.Arnold, M. S., et al., J. Phys. Chem. B (2003) 107(3), 659ini, E., et al., Appl. Phys. Lett.(2002) 81(10), 18694.Bai, X. D., et al., Appl. Phys. Lett.(2003) 82(26), 48065.Hughes, W. L., and Wang, Z. L., Appl. Phys. Lett.(2003) 82(17), 28866.Shi, L., et al., Appl. Phys. Lett.(2004) 84(14), 26387.Kong, X. Y., and Wang, Z. L., Nano Lett.(2003) 3(12), 16258.Kong, X. Y., et al., Science (2004) 303, 13489.Wang, Z. L., and Kang, Z. C., Functional and Smart Materials – StructuralEvolution and Structure Analysis , Plenum Press, New York, (1998)10.Gao, P. X., and Wang, Z. L., J. Phys. Chem. B (2002) 106(49), 1265311.Gao, P. X., and Wang, Z. L., Appl. Phys. Lett.(2004) 84(15), 288312.Liu, C., et al., Adv. Mater.(2003) 15(10), 83813.Bai, X. D., et al., Nano Lett.(2003) 3(8), 114714.Yang, P. D., et al., Adv. Funct. Mater.(2002) 12(5), 32315.Zhao, Q. X., et al., Appl. Phys. Lett.(2003) 83(1), 16516.Gao, P. X., et al., Nano Lett.(2003) 3(9), 131517.Park, W. I., et al., Appl. Phys. Lett.(2002) 80(22), 423218.Harnack, O., et al., Nano Lett.(2003) 3(8), 109719.Huang, Z. P., et al., Appl. Phys. Lett.(2003) 82(3), 46020.Wang, X. D., et al., Nano Lett.(2004) 4(3), 42321.Wang, X. D., et al., Adv. Mater.(2004), in press 22.Wang, X. D., et al., J. Phys. Chem. B (2004), in press23.Gao, P. X., and Wang, Z. L., J. Am. Chem. Soc.(2003) 125(37), 11299Fig. 8 Single-crystalline, polyhedral cages and shells of ZnO.。
纳米氧化锌(性能表征、形态、表面改性)
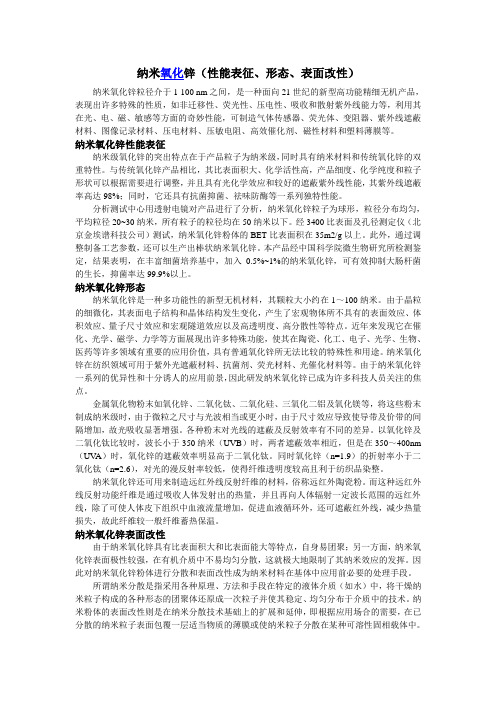
纳米氧化锌(性能表征、形态、表面改性)纳米氧化锌粒径介于1-100 nm之间,是一种面向21世纪的新型高功能精细无机产品,表现出许多特殊的性质,如非迁移性、荧光性、压电性、吸收和散射紫外线能力等,利用其在光、电、磁、敏感等方面的奇妙性能,可制造气体传感器、荧光体、变阻器、紫外线遮蔽材料、图像记录材料、压电材料、压敏电阻、高效催化剂、磁性材料和塑料薄膜等。
纳米氧化锌性能表征纳米级氧化锌的突出特点在于产品粒子为纳米级,同时具有纳米材料和传统氧化锌的双重特性。
与传统氧化锌产品相比,其比表面积大、化学活性高,产品细度、化学纯度和粒子形状可以根据需要进行调整,并且具有光化学效应和较好的遮蔽紫外线性能,其紫外线遮蔽率高达98%;同时,它还具有抗菌抑菌、祛味防酶等一系列独特性能。
分析测试中心用透射电镜对产品进行了分析,纳米氧化锌粒子为球形,粒径分布均匀,平均粒径20~30纳米,所有粒子的粒径均在50纳米以下。
经3400比表面及孔径测定仪(北京金埃谱科技公司)测试,纳米氧化锌粉体的BET比表面积在35m2/g以上。
此外,通过调整制备工艺参数,还可以生产出棒状纳米氧化锌。
本产品经中国科学院微生物研究所检测鉴定,结果表明,在丰富细菌培养基中,加入0.5%~1%的纳米氧化锌,可有效抑制大肠杆菌的生长,抑菌率达99.9%以上。
纳米氧化锌形态纳米氧化锌是一种多功能性的新型无机材料,其颗粒大小约在1~100纳米。
由于晶粒的细微化,其表面电子结构和晶体结构发生变化,产生了宏观物体所不具有的表面效应、体积效应、量子尺寸效应和宏观隧道效应以及高透明度、高分散性等特点。
近年来发现它在催化、光学、磁学、力学等方面展现出许多特殊功能,使其在陶瓷、化工、电子、光学、生物、医药等许多领域有重要的应用价值,具有普通氧化锌所无法比较的特殊性和用途。
纳米氧化锌在纺织领域可用于紫外光遮蔽材料、抗菌剂、荧光材料、光催化材料等。
由于纳米氧化锌一系列的优异性和十分诱人的应用前景,因此研发纳米氧化锌已成为许多科技人员关注的焦点。
纳米氧化锌
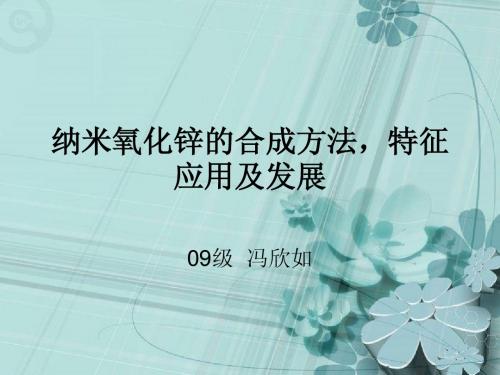
其反应方程式:
ZnO---OH +
纳米氧化锌的合成方法,特征 应用及发展
09级 冯欣如
简介:纳米氧化锌具有纳米材料和优质半导体材料两 方面功能,是一种新型的直接宽带隙半导体材料,在液 晶显示器、太阳能电池、保护性涂层、气敏元件、固 体照明光源和紫外发光器等领域表现出潜在应用前景
太阳能电池板
液晶显示器
一.纳米ZnO合成方法: 纳米氧化锌的制备方法有物理方法和化学方法。物理 方法是将常规的粉体经机器粉碎,球磨而制。化学方法是 从原子或分子,成核 , 生成纳米级的超导微粒 物理合成方法 合 成 方 法 1.气相反映法 化学合成方法 2.液相反映法 3.固相反映法
3.紫外一可见光分析
样品浓度均为0.1g/L纳米氧化锌的禁带宽度为3.2eV,它所对应的吸收 波长为388nn ,可以看出,在290一400nm紫外光区离子交换树脂一步 法制备的纳米氧化锌和离子交换树脂一水热法制备的纳米氧化锌的吸光 度大小相当,曲线走势相同,两者均具有明显较高的吸光度
不同合成方法的纳米氧化锌的紫外一可见光分析图
二.纳米ZnO性质表征
纳米氧化锌的性质表征主要通过:电镜,热重 ,XRD,红外光谱 紫外一可见光等分析方法从不同的角度来分析纳米ZnO的表征 1.电镜分析法
可以看出,生成的产物呈形貌均一的棒状结构,直径约为80一100nm的 一维氧化锌纳米棒
离子交换树脂法
水热法
2.红外光谱分析 如图,523cm-1处为氧化锌的特征峰,带形尖锐,说明产物中存在大量
纳米氧化锌晶体概述

纳米氧化锌晶体概述作者姓名:00班级:00学号:*********联系方式:000000000000****************纳米氧化锌晶体概述钱学森91 马博摘要:纳米氧化锌是一种具有特异性能并且用途广泛的新材料,同时也是一种重要的基础化工原料。
本文首先介绍了纳米氧化锌晶体的基本物理和化学性质,基于这些性质,进一步阐述了纳米氧化锌在各个行业的应用。
其次,本文对纳米氧化锌的制备方法进行了较为详细和系统的介绍。
于此同时,为了对纳米氧化锌的性质进行改进,以扩大其应用领域,最后,我们又对纳米氧化锌的表面改型进行了较为深入地分析。
关键词:纳米ZnO;性质;应用;制备;改性目录1 纳米氧化性概述 (5)1.1氧化锌的基本性质 (5)1.2氧化锌晶体的结构 (5)1.3纳米氧化锌的基本性能[3] (5)1.3.1表面效应 (5)1.3.2体积效应 (5)1.3.3量子尺寸效应 (6)1.3.4宏观量子隧道效应 (6)2 纳米氧化锌的应用 (6)2.1纳米氧化锌在橡胶轮胎中的应用[6] (6)2.2纳米氧化锌在陶瓷中的应用[8] (6)2.3纳米氧化锌在防晒化妆品中的应用 (6)2.4纳米氧化锌在油漆涂料中的应用 (7)2.5纳米氧化锌在纺织中的应用 (7)2.6纳米氧化锌在催化剂和光催化剂中的应用 (7)2.7纳米氧化锌在磁性材料中的应用[5] (7)2.8作为填充剂的应用 (8)3 纳米氧化锌的制备方法 (8)3.1固相法 (8)3.1.1燃烧法[14] (8)3.1.2固相合成法[14] (8)3.2液相法 (8)3.2.1直接沉淀法 (8)3.2.2均匀沉淀法[16] (9)3.2.3并流沉淀法[17] (9)3.2.4溶胶-凝胶法[18] (9)3.2.5水热合成法[19] (10)3.2.6微乳液法[20] (10)3.3气相法[21,22] (10)3.3.1激光诱导气相沉积法 (10)3.3.2气相反应合成法 (10)3.3.3喷雾热解法 (10)3.3.4化学气相氧化法 (10)4 纳米氧化锌的表面改性 (11)4.1表面物理修饰法 (11)4.1.1表面活性剂法[24] (11)4.1.2表面沉积法 (11)4.2表面化学修饰法 (11)4.2.1酯化反应法[27] (11)4.2.2 偶联剂法[24] (11)4.2.3表面接枝改性法[28] (12)4.2.4 机械化学修饰[29] (12)4.2.5外层膜修饰 (12)4.2.6 高能量表面修饰 (12)4.2.7其它方法[30] (13)1 纳米氧化性概述1.1 氧化锌的基本性质氧化锌,俗称锌白,属六方晶系纤锌矿结构,白色或浅黄色晶体或粉末,无毒,无臭,系两性氧化物,不溶于水和乙醇,溶解于强酸和强碱,在空气中能吸收二氧化碳和水[1]。
- 1、下载文档前请自行甄别文档内容的完整性,平台不提供额外的编辑、内容补充、找答案等附加服务。
- 2、"仅部分预览"的文档,不可在线预览部分如存在完整性等问题,可反馈申请退款(可完整预览的文档不适用该条件!)。
- 3、如文档侵犯您的权益,请联系客服反馈,我们会尽快为您处理(人工客服工作时间:9:00-18:30)。
一维形式
目前,ZnO 一维纳米材料及其纳米结构的合成方法 主要有化学气相沉积、基于VLS 机理的催化生长 以及磁控溅射法等气相法以及模板辅助合成、电 化学沉积 和溶液生长等液相法。与设备昂贵且能 耗高的气相法相比,液相法合成ZnO 一维纳米材料 具有设备简单以及合成温度低的特点。其中,不需 借助任何模板、表面活性剂以及外加电场的溶液 生长法更是具有容易调控材料尺寸、成本低且便 于大规模化的优势 。因此,近年来,溶液生长ZnO 一维纳米材料并构筑其复合纳米结构的研究成为 了国际热点研究课题
17
n 纳米ZnO粉体(零维) n 纳米ZnO阵列(一维)
固相法、气相法、液相法。
n 纳米ZnO薄膜(二维)
n 纳米ZnO晶体(三维) 固相法制备纳米氧化锌的原理是将两
种物质分别研磨、混合后,充分研磨 气相得法到可前分驱为物物,理再气加相热沉分积解法得、到脉纳冲米激氧光沉 积法、化学气相传化输锌氧粉化末法。等。气相生长法 制得的纳米氧化锌粒径小、产品分散性好,
3
a.岩盐矿结构 b.闪锌矿结构 c.六方纤锌矿 结构
4
n 体积效应 n 表面效应 n 量子尺寸效应 n 宏观量子隧道效应 n 界面相关效应 n 介电限域效应
微粒分散在异质介质中由于界面 能的存在,引起体系介电性能增强 的现象。当微粒的折射率和介质 的折射率相差很大,微粒表面和内 部的场强比入射场强显著增加,引 起的局部场强增加的现象就是介 电限域效应。这种纳米微粒的介 电限域效应对材料的光吸收、光 学非线性、光化学性能等有非常 重要的影响。
14
二维形式
15
三维形式
自从报导用热蒸发法合成了ZnO 纳米晶粒自组装 的多面笼、球壳结构以来, 许多研究人员相继报导 了各自在不同的实验条件下用热蒸发法合成的 ZnO 微纳米空心球结构。合成的ZnO 纳米晶粒自 组装的多面笼、球壳的SEM图像, 是Lu和L iao等 人合成的内外表面生长有纳米线的ZnO 空心微球 的SEM图像
9
液相法
• 液相法:制备纳米微粒是将均相溶液通过 各种途径使溶质和溶剂分离, 溶质形成一定 形状和大小的颗粒, 得到所需粉末的前驱体, 热解后得到纳米微粒。
• 优缺点:液相法具有设备简单、原料容易 获得、纯度高、均匀性好、化学组成易于 控制等优点。液相法包括沉淀法、水解法 、水热法、微乳液法、溶胶- 凝胶法等, 其 中应用最广的是溶胶- 凝胶法和沉淀法。
16
复合形式
纳米氧化锌的导热性能明显优于炭黑和白 炭黑等传统补强填料,其对EPDM具有较好的 补强作用,纳米氧化锌/EPDM复合材料的生热 较低;采用偶联剂Si69对纳米氧化锌进行原位 改性可以改善纳米氧化锌粒子与EPDM间的 界面作用,提高其分散性,从而显著提高复合 材料的物理性能,降低生热;改性纳米氧化锌 /EPDM复合材料的物理性能和导热性能良好, 可用于动态工况下使用的橡胶制品。
维持在狭小的范围内。
19
水热法制纳米粉
掺杂物质
硝酸
混合溶液
六水合硝酸锌 乙醇胺 聚乙二醇
蒸干多余硝酸 搅拌均匀 透明溶液 反应釜反应
离心、分离
退火
20
n 纳米ZnO粉体(零维) n 纳米ZnO阵列(一维) n 纳米ZnO薄膜(二维) n 纳米ZnO晶体(三维)
10
气相法
• 气相法:指直接利用气体或者通过各 种手段将物质变为气体, 使之在气体状 态下发生物理或化学反应, 最后在冷却 过程中凝聚长大形成纳米微粒的方法 。
• 气相法包括溅射法、化学气相反应法 、化学气相凝聚法、等离子体法、激 光气相合成法、喷雾热分解法等。
11
纳米氧化锌材料的分类
按结构形 式分
开题报告
之
纳米氧化锌
成员:
组长: 2020/4/24
主要内容
简介 分类 合成方法 应用 现状与发展
2
第三代半导体材料 禁带宽度:3.37eV
纯氧化锌是 N型半导体
ZnO的激子束缚 能为60meV
Ø 又称宽禁带半导体或高温半导体 Ø SiC,GaN,ZnO,AlN,金刚石 Ø 很多优异的性能
Ø 晶体中有填隙原子Zn和氧空位缺陷, 锌是浅能级缺陷氧空位是神能级缺陷
5
其晶格中可能产生的 本征点缺陷有6 种: 氧空位、锌空位、反 位氧、反位锌、氧填 隙以及锌填隙。从能 级角度分类,点缺陷 可分为浅能级缺陷和 深能级缺陷, 其中深 能级对氧化锌的光学 性质影响较大。研究 认为, 位于465~ 520nm 的蓝-绿可见 发光带主要是氧化锌 的深能级缺陷引起的 。
6
目的:改善性能
压电性能 光学性能 气敏特性 电学性能 催化性能
杂质: 稀土、铝、锡、相法
液相法
气相法
8
固相法
• 固相法:是将金属盐或金属氧化物按一 定比例充分混合、研磨后进行煅烧, 通过发 生固相反应直接制得纳米粉末。
• 优缺点:运用固相法制备纳米ZnO 具有 操作和设备简单安全, 工艺流程短等优点, 所以工业生产前景比较乐观, 其不足之处是 制备过程中容易引入杂质, 纯度低, 并且容 易使金属氧化, 颗粒不均匀以及形状难以控 制
反应条件易控制,易得到均匀超细粒子,缺
点是产物中有原料残存,工艺技术较复杂, 成本高,一次性投资大。
18
n 直接沉淀法 n 均匀沉淀法 n 水热合成法 n 溶胶——凝胶法 n 超声波合成法 n 喷雾热分解法
沉淀物颗粒晶型成整且致密,避 免了杂志的共沉淀,粒子的粒径 分布均匀,分散性好。反应条件 温和,易于洗涤,工业前景好, 但由于Zn(OH)2的两性,PH必须
零·一维形 式
二·三维形 式
复合形式
12
零维形式
• 金属氧化物粉末如氧化锌、二 氧化钛、二氧化硅、三氧化二 铝及氧化镁等,将这些粉末制 成纳米级时,由于微粒之尺寸 与光波相当或更小时,由于尺 寸效应导致使导带及价带的间 隔增加,故光吸收显著增强。 各种粉末对光线的遮蔽及反射 效率有不同的差异。以氧化锌 及二氧化钛比较时,波长小于 350纳米(UVB)时,两者遮 蔽效率相近,但是在350~ 400nm(UVA)时,氧化锌的 遮蔽效率明显高于二氧化钛。 同时氧化锌(n=1.9)的折射率 小于二氧化钛(n=2.6),对光 的漫反射率较低,使得纤维透 明度较高且利于纺织品染整。