WO3纳米材料的H2S气敏特性
wo3-乙醇气敏材料
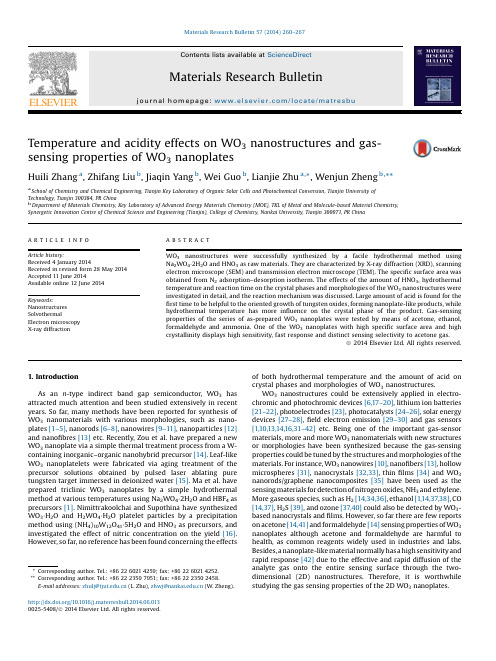
Temperature and acidity effects on WO 3nanostructures and gas-sensing properties of WO 3nanoplatesHuili Zhang a ,Zhifang Liu b ,Jiaqin Yang b ,Wei Guo b ,Lianjie Zhu a ,*,Wenjun Zheng b ,**aSchool of Chemistry and Chemical Engineering,Tianjin Key Laboratory of Organic Solar Cells and Photochemical Conversion,Tianjin University of Technology,Tianjin 300384,PR China bDepartment of Materials Chemistry,Key Laboratory of Advanced Energy Materials Chemistry (MOE),TKL of Metal and Molecule-based Material Chemistry,Synergetic Innovation Centre of Chemical Science and Engineering (Tianjin),College of Chemistry,Nankai University,Tianjin 300071,PR ChinaA R T I C L E I N F OArticle history:Received 4January 2014Received in revised form 28May 2014Accepted 11June 2014Available online 12June 2014Keywords:Nanostructures SolvothermalElectron microscopy X-ray diffractionA B S T R A C TWO 3nanostructures were successfully synthesized by a facile hydrothermal method using Na 2WO 4Á2H 2O and HNO 3as raw materials.They are characterized by X-ray diffraction (XRD),scanning electron microscope (SEM)and transmission electron microscope (TEM).The speci fic surface area was obtained from N 2adsorption –desorption isotherm.The effects of the amount of HNO 3,hydrothermal temperature and reaction time on the crystal phases and morphologies of the WO 3nanostructures were investigated in detail,and the reaction mechanism was rge amount of acid is found for the first time to be helpful to the oriented growth of tungsten oxides,forming nanoplate-like products,while hydrothermal temperature has more in fluence on the crystal phase of the product.Gas-sensing properties of the series of as-prepared WO 3nanoplates were tested by means of acetone,ethanol,formaldehyde and ammonia.One of the WO 3nanoplates with high speci fic surface area and high crystallinity displays high sensitivity,fast response and distinct sensing selectivity to acetone gas.ã2014Elsevier Ltd.All rights reserved.1.IntroductionAs an n -type indirect band gap semiconductor,WO 3has attracted much attention and been studied extensively in recent years.So far,many methods have been reported for synthesis of WO 3nanomaterials with various morphologies,such as nano-plates [1–5],nanorods [6–8],nanowires [9–11],nanoparticles [12]and nano fibres [13]etc .Recently,Zou et al.have prepared a new WO 3nanoplate via a simple thermal treatment process from a W-containing inorganic –organic nanohybrid precursor [14].Leaf-like WO 3nanoplatelets were fabricated via aging treatment of the precursor solutions obtained by pulsed laser ablating pure tungsten target immersed in deionized water [15].Ma et al.have prepared triclinic WO 3nanoplates by a simple hydrothermal method at various temperatures using Na 2WO 4Á2H 2O and HBF 4as precursors [1].Nimittrakoolchai and Supothina have synthesized WO 3ÁH 2O and H 2WO 4ÁH 2O platelet particles by a precipitation method using (NH 4)10W 12O 41Á5H 2O and HNO 3as precursors,and investigated the effect of nitric concentration on the yield [16].However,so far,no reference has been found concerning the effectsof both hydrothermal temperature and the amount of acid on crystal phases and morphologies of WO 3nanostructures.WO 3nanostructures could be extensively applied in electro-chromic and photochromic devices [6,17–20],lithium ion batteries [21–22],photoelectrodes [23],photocatalysts [24–26],solar energy devices [27–28],field electron emission [29–30]and gas sensors [1,10,13,14,16,31–42]etc.Being one of the important gas-sensor materials,more and more WO 3nanomaterials with new structures or morphologies have been synthesized because the gas-sensing properties could be tuned by the structures and morphologies of the materials.For instance,WO 3nanowires [10],nano fibers [13],hollow microspheres [31],nanocrystals [32,33],thin films [34]and WO 3nanorods/graphene nanocomposites [35]have been used as the sensing materials for detection of nitrogen oxides,NH 3and ethylene.More gaseous species,such as H 2[14,34,36],ethanol [1,14,37,38],CO [14,37],H 2S [39],and ozone [37,40]could also be detected by WO 3-based nanocrystals and films.However,so far there are few reports on acetone [14,41]and formaldehyde [14]sensing properties of WO 3nanoplates although acetone and formaldehyde are harmful to health,as common reagents widely used in industries and labs.Besides,a nanoplate-like material normally has a high sensitivityand rapid response [42]due to the effective and rapid diffusion of the analyte gas onto the entire sensing surface through the two-dimensional (2D)nanostructures.Therefore,it is worthwhile studying the gas sensing properties of the 2D WO 3nanoplates.*Corresponding author.Tel.:+862260214259;fax:+862260214252.**Corresponding author.Tel.:+862223507951;fax:+862223502458.E-mail addresses:zhulj@ (L.Zhu),zhwj@ (W.Zheng)./10.1016/j.materresbull.2014.06.0130025-5408/ã2014Elsevier Ltd.All rights reserved.Materials Research Bulletin 57(2014)260–267Contents lists available at ScienceDirectMaterials Research Bulletinj o u r n a l h o m e p a g e :w w w.e l s e v i e r.c o m /l o c a t e /m a t r e s buHerein,a facile hydrothermal route was adopted for syntheses of series of WO3nanostructures.The effects of the amount of HNO3,hydrothermal temperature and reaction time on crystal phases and morphologies of WO3products were investigated and discussed.The formation mechanism of the WO3nanoplates was studied on the basis of control experiments.The gas-sensing properties of the as-prepared WO3on acetone,ethanol,formalde-hyde and ammonia were evaluated,and a nanoplate-like WO3 shows a high sensitivity,rapid response and distinct selectivity to acetone gas.2.Experimental2.1.Synthesis of WO3nanostructuresIn a typical procedure,0.3299g of Na2WO4Á2H2O(1mmol)was added into certain volume of distilled water and dissolved thoroughly under stirring.Then,certain amount of HNO3(w, 65%)was dropped into the solution and kept stirring for10min. The total volume of the solution was always kept to be25.0mL.The above mixture was transferred into a33.0mL of Teflon-lined stainless steel autoclave,sealed and kept in an electric oven at certain temperature for12h.The autoclave was then taken out and allowed to cool naturally to room temperature.The obtained precipitate was separated by centrifugation,washed by distilled water and absolute ethanol for three times,respectively,and dried at50 C.2.2.CharacterizationsThe XRD measurements were performed on a Rigaku D/max-2500diffractometer with Cu K a radiation(l=0.154056nm)at 40kV and100mA.The SEM images were taken with a JEOL JSM-6700F scanning electron microscope.The TEM and high resolution TEM(HRTEM)images of the WO3nanoplates were obtained on a JEOL JEM-2100F microscope operating at200kV.The N2adsorp-tion–desorption isotherm measurements were operated on a V-Sorb2800P surface area and pore size analyzer.2.3.Fabrication and test of WO3gas sensorsThe schematic illustration of the gas-sensing measurement and working principle of HW-30A system can be found in the references[1,43].The WO3nanoplates were slightly grinded with several drops of terpineol in an agate mortar.The formed slurry was coated onto an alumina tube with a diameter of1mm and length of4mm,positioned with a pair of Au electrodes and four Pt wires on both ends of the tube.After calcination at400 C for1h, the alumina tube was jointed with the measuring electric circuit.In order to improve the stability and repeatability,the gas-sensing element was aged at340 C for two weeks before the measure-ment.Acetone,ethanol,formaldehyde and ammonia with various concentrations were chosen to evaluate the gas-sensing properties of the as-prepared WO3.The sensor response is defined as S=R a/R g, where R a and R g are the electrical resistance of the sensor in air and in test gas,respectively.The response and recovery time is defined as the time for the sensor to reach90%of its maximum response and fall to10%of its maximum response,respectively.3.Results and discussion3.1.Effects of hydrothermal temperature and the amount of HNO3on the crystal phases and morphologies of the productsFig.1shows the crystal phase evolution of the products obtained at120 C using various amount of HNO3.When0.5mL of HNO3is used,the main product is orthorhombic WO3ÁH2O(JCPDS 43-0679)with a little impurity(Fig.1a).Increasing the amount of HNO3to1.5mL leads to the formation of a pure orthorhombic WO3ÁH2O(Fig.1b).The monoclinic phase of unhydrous WO3 (JCPDS43-1035)appears when2.5mL of HNO3is used and the relative peak intensity of this phase increases gradually by increasing the volume of HNO3until12.0mL(Fig.1c–f).When the amount of HNO3is further increased,however,no pure phase of monoclinic WO3is produced(Fig.1g and h).On the contrary,the relative intensity of the diffraction peaks of the monoclinic WO3 becomes lower,but that of the WO3ÁH2O phase becomes higher again.When the volume of HNO3reaches20.0mL,the diffraction peaks of the monoclinic WO3phase become even faint.The morphology evolution of the products prepared at120 C using various amount of HNO3is demonstrated in Fig.2.It was observed that the product’s morphology changes greatly in the low volume region of HNO3.A nanoparticle and nanorod mixture was collected when0.5mL of HNO3was used in the reaction system. Slightly increasing the volume of HNO3to1.5mL would lead to the formation of a bigflower-like hierarchical structure which is composed of many crossed and lapped quasi-round nanoplates with rough surfaces.The accumulated quasi-round nanoplates start dispersal and the surfaces become smooth when the volume of HNO3is increased to2.5mL.Most of the nanoplates are about 600nm in diameter and25nm in thickness.If5.0mL of HNO3is used,square-like nanoplates with a side length of around200nm and thickness of30–40nm are obtained.The surfaces of these nanoplates are rather smooth.When the volume of HNO3is in the range of 5.0–12.0mL,the products’morphologies are similar, unless the size of the nanoplate gradually becomes smaller with increasing the acid quantity.Some square-like nanoplates become a bit irregular and their surface roughness also increases if the amount of HNO3reaches15.0mL.Further increasing the amount of HNO3to20.0mL,irregularly shaped nanoplates with rather rough surfaces were obtained.This morphology is similar to that of the product in Fig.2c,unless the size is much smaller.One may notice that the phase composition of these two products(Fig.1c and h)is also similar.The other series of products were obtained at180 C,a higher hydrothermal temperature.The corresponding XRD patterns are shown in Fig.3.Orthorhombic WO3ÁH2O(JCPDS43–0679)was produced using0.5mL of HNO3.Increasing the amount of HNO3to 1.5mL leads to the formation of a mixture with most orthorhombic WO3ÁH2O and a little monoclinic WO3(JCPDS43–1035).The phase compositions of the products change dramatically withslightlyFig. 1.XRD patterns of the products prepared by hydrothermal method at120 C using various amount of HNO3:(a)0.5mL,(b)1.5mL,(c)2.5mL,(d)5.0mL,(e) 10.0mL,(f)12.0mL,(g)15.0mL and(h)20.0mL.H.Zhang et al./Materials Research Bulletin57(2014)260–267261increasing the volume of HNO 3from 1.5mL to 2.0mL.Pure monoclinic WO 3is obtained in the presence of 2.0mL of HNO 3.With further increasing the amount of HNO 3,the crystal phases of the products do not change any more.But the intensity of the diffraction peaks increases firstly and then declines slightly.The peak intensity reaches maximum using 10mL of HNO 3,which indicates the highest crystallinity of the WO 3product obtained at this condition.Thus,10mL of HNO 3is a suitable amount to obtain a pure monoclinic WO 3with a high crystallinity.The morphology evolution of the products prepared at 180 C using various amount of HNO 3is demonstrated in Fig.4.When 0.5mL of HNO 3is used,a nanoparticle –nanorod mixture (Fig.4a)is obtained,similar to the morphology of the product prepared at 120 C (Fig.2a).However,in the case of 1.5mL HNO 3,the two products prepared at these two hydrothermal temperatures have completely different morphologies.As shown in Fig.4b,the product comprises many non-uniform big rectangular blocks andplates.Slightly increasing the HNO 3–2.0mL leads to formation of WO 3nanoplates with different size,and some nanoplates accumulate with their large surfaces.When 10.0mL or 15.0mL of HNO 3is used,the product is well-dispersed nanoplates with the large surfaces exposed (Fig.4d and e).The side length and thickness of an individual nanoplate are approximately 100nm and 40nm,respectively.Changing the amount of HNO 3in the range of 10–15mL does not in fluence the morphology of the products.But in case of 20.0mL HNO 3,the uniformity of the product decreases and the size of the nanoplate increases to certain extent,although its crystal phase is kept unchanged.Because both the uniformity and crystallinity of the sample prepared at 180 C using 10.0mL of HNO 3are high,its TEM and HRTEM images were taken.As shown in Fig.5a,the side length and thickness of the most WO 3nanoplates are around 100nm and 30–40nm,respectively,which is consistent with the SEM result.The clear 2D crystal lattice (Fig.5b)shows that theinterplanarFig. 2.SEM images of the products prepared by hydrothermal method at 120 C using various amount of HNO 3:(a)0.5mL,(b)1.5mL,(c)2.5mL,(d)5.0mL,(e)10.0mL,(f)12.0mL,(g)15.0mL and (h)20.0mL.262H.Zhang et al./Materials Research Bulletin 57(2014)260–267distances are 0.37and 0.38nm,corresponding to (020)and (002)crystal planes,respectively.The fast Fourier transform pattern (the inset)indicates its single crystalline nature.On the basis of above results we conclude that both hydrothermal temperature and the amount of HNO 3in fluence the crystal phase and morphology of the product.Fig.6shows a summary of these two effects.Similar to the result in our previous work [1],low temperature favours the formation of WO 3ÁH 2O andhigh temperature is propitious to production of anhydrous WO 3.Increasing temperature results in dehydration of WO 3ÁH 2O,forming pure WO 3phase,even using a small volume of HNO 3(for example,2.0mL HNO 3).However,at the low hydrothermal temperature,120 C,no pure phase of WO 3was obtained even if a large volume of HNO 3was used.In the region of small volume of HNO 3,the crystal phases and morphologies of the products are found more sensitive to the change in acid quantity and reaction temperature.For instance,when 1.5mL of HNO 3was used,a hierarchical flower-like hydrous WO 3was formed at 120 C,but a non-uniform rectangular block and plate-like hydrous and anhydrous WO 3mixture is the main product at 180 C.This may be attributed to different nucleation rate,diffusion rate of nanoparticles and dehydration rate at different temperatures.At high temperature,the formation rate of tungsten oxide nucleus is fast and the hydrous WO 3loses water quickly once beyond its decomposition temperature,which partially counteracts the in fluence of larger amount of water on the crystal phase of the product when small volume of HNO 3is used.Moreover,the diffusion rate of nanoparticles is low at low temperature,which contributes to the formation of a big architecture.But a high diffusion rate at high temperature helps to epitaxy along certain crystal face [44].Therefore,different morphological products were produced at two hydrothermal temperatures using 1.5mL of HNO 3.When large amount of HNO 3(for instance,10mL HNO 3)is used,however,it is different from the above case.The similar morphology of the two products obtained at the twotemperaturesFig. 3.XRD patterns of the products prepared by hydrothermal method at 180 C using various amount of HNO 3:(a)0.5mL,(b)1.5mL,(c)2.0mL,(d)10.0mL,(e)15.0mL and (f)20.0mL.Fig.4.SEM images of the products prepared by hydrothermal method at 180 C using various amount of HNO 3:(a)0.5mL,(b)1.5mL,(c)2.0mL,(d)10.0mL,(e)15.0mL and (f)20.0mL.H.Zhang et al./Materials Research Bulletin 57(2014)260–267263clearly shows that large amount of acid is bene ficial to the oriented growth of the tungsten oxides,forming nanoplates.This,on the one hand,may be related to the high degree of supersaturation of the solution,which will increase the nucleation rate and dehydration rate of H 2WO 4,and ultimately increase the reaction rate.On the other hand,the acidity of a reaction system in fluences surface energies of certain crystal facets [45],which may signi ficantly in fluence the oriented growth of a crystal,and consequently in fluence the morphology of the product.Thus,suitable amount of acid is a dominant factor to the formation of nanoplate-like morphology.However,the acidity effect on the crystal phase of the product is found weaker than that of temperature.The low nucleation rate at 120 C results in the larger size of the nanoplates (compared to the product prepared at 180 C),which accords with the crystallization principle.The low dehydration rate at the lower temperature leads to incomplete dehydration of the product,forming a mixed phase of product.Thus,a larger size of mixed phase of nanoplate was obtained at 120 C using 10mL of HNO 3.3.2.Formation mechanismBecause the product prepared at 180 C using 15.0mL of HNO 3shows the better gas-sensing property,its time-dependent crystal phase and morphology evolutions were investigated.It represents the effect of hydrothermal time on the crystal phase and morphology of a WO 3nanostructure.As shown in Fig.S1and S2in the supporting information (ESI),pure uniform orthorhombic WO 3ÁH 2O (JCPDS 43–0679)nanoplates were obtained at 1h,which were quickly transformed to WO 3nanoplates.A mixture of WO 3ÁH 2O and WO 3was collected at 1.5h and pure monoclinic WO 3(JCPDS 43-1035)nanoplates were produced at 2h.These results con firm that a hydrous WO 3was formed at the early stage of the reaction and prolonging hydrothermal time results in a quick dehydration of WO 3ÁH 2O.The corresponding chemical reactions contain:WO 2À4þ2H þ!WO 3ÁH 2O(1)WO 3ÁH 2O !WO 3þH 2O(2)This reaction process is similar to the work by Lee et al.[46,46],where the high concentrations of HNO 3(0.29–11.5mol L À1)were replaced by low concentrations of HCl (pH 2–6)and NaCl was used as a capping agent.However,WO 3nanorods were obtained in their work although a similar procedure was applied.Lee et al.believed that NaCl selectively adsorbed onto the crystal planes parallel to the c -axes of WO 3crystal nucleus to accelerate the preferential growth,resulting in the formation of 1D structure.In our case,no capping agent or directing agent was used.Besides,as shown in Fig.6,a large amount of acid is bene ficial to the oriented growth of nanoplate-like tungsten oxides.Except the external factors,the intrinsic crystal structure is also an essential factor for morphology control of the product.The orthorhombic H 2WO 4consists of layers of [WO 6]octahedra,which share their four equatorial oxygen atoms.Water molecules and oxygen atoms in the axial positions of the [WO 6]octahedra form hydrogen bonds with the neighboring layers [3],as shown in Fig.S3a in the ESI.The monoclinic WO 3possesses a distorted ReO 3structure,see Fig.S3b.The layers of [WO 6]octahedra in the orthorhombic H 2WO 4and the monoclinic WO 3are similar.The shared four equatorial oxygen atoms extend forming a 2D plane structure.At high temperature,the hydrogen bonds in the layers of [WO 5(OH 2)]octahedra of the H 2WO 4were replaced by W ÀÀO ÀÀW bonds forming a three-dimensional W ÀÀO ÀÀW lattice-work and losing the crystal waters.The similarity of the WO 6octahedral sheets in H 2WO 4and WO 3results in the formation of the nanoplate-like WO 3.3.3.Gas-sensing properties and mechanismAll gas-sensing measurements were operated at 300 C.Therefore,the samples were calcined at 450 C for 2h before the gas-sensing measurements to ensure their thermal stability.The XRD patterns of the calcined WO 3samples are shown in Fig.S4in the ESI.All diffraction peaks for all the samples can be indexedtoFig. 5.(a)TEM and (b)HRTEM images of the sample prepared at 180 C using 10.0mL of HNO 3(the inset is the fast Fourier transformpattern).Fig.6.Schematic representation of the formation of the WO 3nanostructures with various morphologies and crystal phases:(a)orthorhombic WO 3ÁH 2O,(b)a mixture of orthorhombic WO 3ÁH 2O and monoclinic WO 3and (c)monoclinic WO 3,prepared at two hydrothermal temperatures using various amount of HNO 3.264H.Zhang et al./Materials Research Bulletin 57(2014)260–267monoclinic WO3(JCPDS43-1035),which indicates that pureanhydrous WO3products were obtained after the heat treatment.The SEM images of the calcined WO3samples,Fig.S5in the ESI,demonstrate that the product morphologies before and after theheat treatment are similar,which verifies again that thecondensation from WO3ÁH2O to WO3is a topotactic conversion process.The synthetic conditions of the precursors,the morphol-ogies and Brunauer–Emmett–Teller(BET)surface areas of thecalcined WO3products are summarized in Table1.The gas-sensing properties of the series of WO3nanomaterialsarefirst evaluated by means of200ppm acetone gas(Fig.7a).Theresults show that the sensor based on the S3sample is moresensitive than the others.The response of the S2sensor is slightlylower than that of the S3sensor.The third sensitive sensor is basedon the P3sample.The high responses of the S2,S3and P3sensorsmay be attributed to their high BET surface areas,highcrystallinities and plate-like morphologies[38].The most sensitive three sensors based on the samples S2,S3and P3were then chosen to investigate the sensitivities in thepresence of various concentrations of acetone gases,from10ppmto400ppm.As shown in Fig.7b,the S3sensor always has thehigher response than the other two sensors to any concentration ofacetone gas.The responses for all the three sensors increasesignificantly with increasing the concentration of acetone gas.Forinstance,the response for the S3sensor increases from4.1to15.8with increasing the concentration of acetone from10ppm to400ppm.Fig.8a represents the dynamic responses of the S2,S3and P3sensors to acetone gases with various concentrations from10ppmto400ppm.Sharp rises and drops in voltage values were observedfor all concentrations of acetone gases when they were injectedand discharged,respectively.This indicates that the WO3nano-plate sensors have a wide range of acetone-detecting abilities andare of fast response and recovery speeds to acetone gases.Forexample,the response and recovery time of the S3sensor are7sand23s,respectively,for100ppm acetone gas(Fig.8b).The resultsalso show that the response increases with increasing theconcentration of acetone gas at the same operating conditions.The sensing selectivity of the S3sensor was explored bycomparing the responses of the S3sensor to acetone,ethanol,formaldehyde and ammonia gases with various concentrations.Asshown in Fig.9,the responses of the S3sensor to all the four gasesincrease gradually with increasing the concentrations of the gases.However,the order of magnitude of the responses to differentgases is quite different.It is less sensitive to ethanol and almostinsensitive to formaldehyde and ammonia,compared to acetone.Thus,the as-prepared WO3nanoplate(S3)displays a distinctsensing selectivity to acetone gas,which could be explained byconsidering its sensing mechanism.As an n-type semiconductor,the sensing mechanism of theWO3nanoplate could be explained using the classical electrondepletion theory[41,47,48].Oxygen molecules in airfirst physically adsorb on the surface of WO3semiconductor.The O2(ads)capturesan electron from WO3forming OÀ2(ads).At an elevated tempera-ture,the chemically adsorbed O2(ads)molecules can obtainenough energy to capture two electrons or OÀ2(ads)to capture one more electron forming OÀ(ads).Because the oxygen ionosorption depletes electrons in the conduction band,conductance of the WO3 sensor is decreased.When the WO3nanoplate sensor is exposed to the reducing gases,acetone,ethanol,formaldehyde and ammonia, the gases react with the OÀ(ads)on the surface of WO3.Referring to the references[49,50],the reactions could be expressed by the following Eqs.(3)–(6).CH2Oþ2OÀðadsÞ!CO2þH2Oþ2eÀ(3) C2H5OHþ6OÀðadsÞ!2CO2þ3H2Oþ6eÀ(4) CH3COCH3þ8OÀðadsÞ!3CO2þ3H2Oþ8eÀ(5) NH3þ5=2OÀðadsÞ!NOþ3=2H2Oþ5=2eÀ(6) The gas removes chemisorbed oxygen anions and releases electrons to the conduction band.This will decrease the amount of the surface OÀ(ads)and increase the concentration of electrons in the conduction band,which eventually increases the conductance of the WO3sensor.According to the Eqs.(3)–(5),more electrons are released with increasing carbon chain.Consequently,the response increases because of the increased conductance of the sensor. Therefore,the gas response of acetone is higher than those of ethanol and formaldehyde gases at the equivalent concentration.As it is known that WO3is an acid oxide and generally it shows relatively high response to ammonia[13,41,51,52],but this is not always the case.For instance,Yu et al.reported that the gas responses of the WO3nanorods are7.6,18and27.5for1mL of ammonia,ethanol and acetone,respectively[35].A similar result was also found by Li and his co-workers[53],where the gas responses of the WO3hollow-spheres are2.89,6.14and13.50for 500ppm of ammonia,ethanol and acetone gases,respectively. These works show that the responses of the WO3nanostructures to ammonia gases are low and much lower than those of ethanol and acetone gases at the equivalent condition,which is similar to our result.Wefind that the response of the as-prepared WO3nanoplate to the alkaline gas,NH3,is lower than those of ethanol and acetone gases although it is slightly higher than that of formaldehyde.One may notice that the four gas responses(Fig.9)of the WO3 nanoplate increase with increasing the quantity of the released electrons(Eqs.3–6).This further confirms the proposed sensing mechanism above.From this point of view,it is reasonable that the as-prepared WO3nanoplate shows a distinct sensing selectivity to acetone gas.The sensing performance of a material can be strongly affected by a few factors.The higher the BET surface area is,the higher the oxygen-adsorption quantity is,and the higher sensitivity the WO3 sensor has[54].High crystallinity is also beneficial to highTable1The synthetic conditions of the precursors and properties of the WO3products after calcination at450 C for2h.Samples T( C)HNO3(mL)[H+](mol LÀ1)Morphologies Surface areas(m2gÀ1)P1120 5.0 2.9Nanoplate17.6P212010.0 5.8Nanoplate24.2P312012.0 6.9Nanoplate28.7P412015.08.6Nanoplate25.3S1180 2.0 1.2Big block and plate12.2S218010.0 5.8Nanoplate23.9S318015.08.6Nanoplate40.1S418020.011.5Nanoplate19.4H.Zhang et al./Materials Research Bulletin57(2014)260–267265sensitivity of a WO 3sensor [38].Therefore,the S3sensor has the highest value of acetone response due to the largest BET surface area exposed to gas and good crystallinity.Besides,the plate-like morphology of the WO 3allows for rapid diffusion of acetone molecules,which results in a fast gas-sensing response.In a word,the WO 3nanoplate (S3)is very promising for fabrication of an acetone gas sensor because of its high sensitivity,distinct selectivity and fast response to acetone gas.4.ConclusionsSeries of tungsten oxide nanostructures with different crystal phases and morphologies have been synthesized at two temper-atures by a facile hydrothermal method using various amount of HNO 3,and the formation mechanism of the WO 3nanoplate was explored based on series of time-dependant control experiments.The results show that the tungstate first reacts with hydrion forming hydrous WO 3,followed by a dehydration rge amount of acid,high temperature and long reaction time are found generally bene ficial to the formation of anhydrous WO 3nano-plates.It is worth mentioning that large amount of acid is found helpful to the oriented growth of the tungsten oxides,forming a nanoplate-like product.But the effect of acid quantity on the crystal phase of a product is weaker than that of temperature.The low dehydration rate at the low temperature (120 C)leads to incompletely dehydration of the product,forming a WO 3ÁH 2O and WO 3mixed phase of nanoplate,even if a large amount of acid (10mL)is used.At the high hydrothermal temperature (180 C)the nucleation rate,diffusion rate of nanoparticles and dehydration rate are increased signi ficantly,resulting in the formation of pure monoclinic WO 3nanoplates,even if a small amount of acid (2mL)is used.The gas-sensing measurements show that the sensitivities of the sensors based on the S3,S2and P3samples are the top three in the presence of 200ppm acetone gas.The S3sensor shows a distinct selectivity and fast response to acetone gas,which implies that the WO 3nanoplate (S3)is a promising material for fabrication of an acetone gassensor.Fig.7.Response comparison of the sensors based on the samples (a)P1-P4and S1-S4in the presence of 200ppm acetone gas and (b)P3,S2and S3in the presence of various concentrations of acetonegases.Fig.8.(a)Dynamic response –recovery curves of the P3,S2and S3sensors for acetone gas detection and (b)response transients of the S3sensor to 100ppm acetone gas.R e s p o n s eConce ntration /pp mFig.9.Sensing selectivity of the S3sensor to four gases with variousconcentrations.266H.Zhang et al./Materials Research Bulletin 57(2014)260–267。
WO3光电催化材料的电子结构及催化性能研究
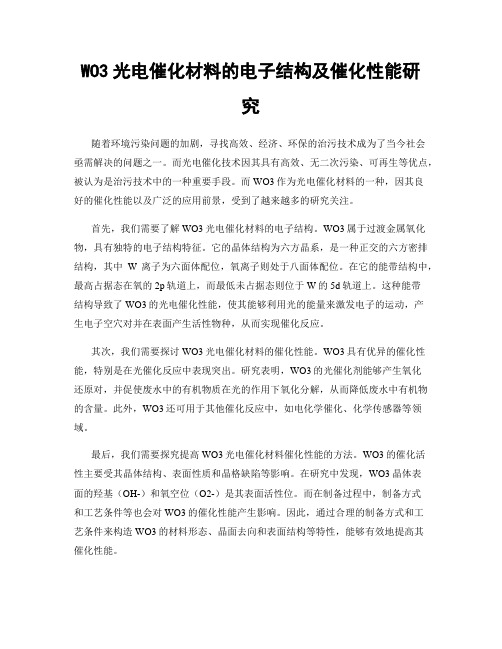
WO3光电催化材料的电子结构及催化性能研究随着环境污染问题的加剧,寻找高效、经济、环保的治污技术成为了当今社会亟需解决的问题之一。
而光电催化技术因其具有高效、无二次污染、可再生等优点,被认为是治污技术中的一种重要手段。
而WO3作为光电催化材料的一种,因其良好的催化性能以及广泛的应用前景,受到了越来越多的研究关注。
首先,我们需要了解WO3光电催化材料的电子结构。
WO3属于过渡金属氧化物,具有独特的电子结构特征。
它的晶体结构为六方晶系,是一种正交的六方密排结构,其中W离子为六面体配位,氧离子则处于八面体配位。
在它的能带结构中,最高占据态在氧的2p轨道上,而最低未占据态则位于W的5d轨道上。
这种能带结构导致了WO3的光电催化性能,使其能够利用光的能量来激发电子的运动,产生电子空穴对并在表面产生活性物种,从而实现催化反应。
其次,我们需要探讨WO3光电催化材料的催化性能。
WO3具有优异的催化性能,特别是在光催化反应中表现突出。
研究表明,WO3的光催化剂能够产生氧化还原对,并促使废水中的有机物质在光的作用下氧化分解,从而降低废水中有机物的含量。
此外,WO3还可用于其他催化反应中,如电化学催化、化学传感器等领域。
最后,我们需要探究提高WO3光电催化材料催化性能的方法。
WO3的催化活性主要受其晶体结构、表面性质和晶格缺陷等影响。
在研究中发现,WO3晶体表面的羟基(OH-)和氧空位(O2-)是其表面活性位。
而在制备过程中,制备方式和工艺条件等也会对WO3的催化性能产生影响。
因此,通过合理的制备方式和工艺条件来构造WO3的材料形态、晶面去向和表面结构等特性,能够有效地提高其催化性能。
综上所述,WO3光电催化材料具有优异的电子结构和催化性能,具有广泛的应用前景。
同时,在研究中提高其催化性能的方法也越来越多。
相信在不久的将来,WO3光电催化材料将在各种治污领域中发挥出更加重要的作用,为我们创造一个更加美好的生活环境。
永磁同步电机,等效磁路法,工作特性曲线
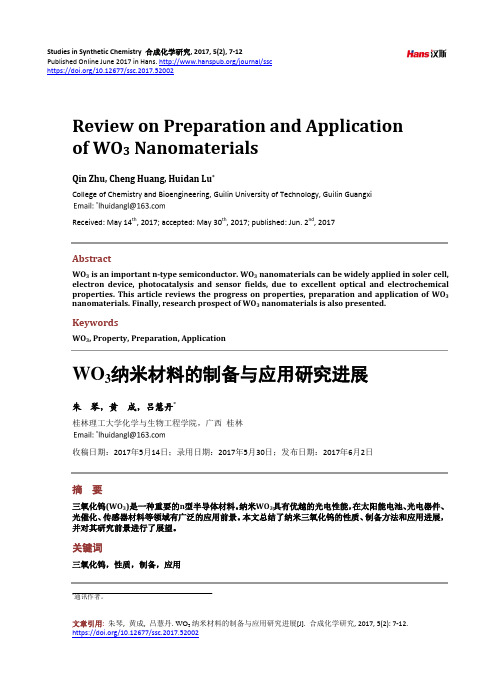
Studies in Synthetic Chemistry 合成化学研究, 2017, 5(2), 7-12Published Online June 2017 in Hans. /journal/sschttps:///10.12677/ssc.2017.52002Review on Preparation and Applicationof WO3 NanomaterialsQin Zhu, Cheng Huang, Huidan Lu*College of Chemistry and Bioengineering, Guilin University of Technology, Guilin GuangxiReceived: May 14th, 2017; accepted: May 30th, 2017; published: Jun. 2nd, 2017AbstractWO3 is an important n-type semiconductor. WO3 nanomaterials can be widely applied in soler cell, electron device, photocatalysis and sensor fields, due to excellent optical and electrochemical properties. This article reviews the progress on properties, preparation and application of WO3 nanomaterials.Finally, research prospect of WO3 nanomaterials is also presented.KeywordsWO3, Property, Preparation, ApplicationWO3纳米材料的制备与应用研究进展朱琴,黄成,吕慧丹*桂林理工大学化学与生物工程学院,广西桂林收稿日期:2017年5月14日;录用日期:2017年5月30日;发布日期:2017年6月2日摘要三氧化钨(WO3)是一种重要的n型半导体材料。
气敏材料的制备及其气敏性能研究

气敏材料的制备及其气敏性能研究随着人类社会的发展,环境污染问题日益突显,如何对环境进行有效的监控和治理成为了亟待解决的问题。
其中,气体污染监测是环境监测的重要分支,而气敏材料的研究及其应用在气体污染监测方面具有重要意义。
气敏材料是一类能对某种气体或气体混合物产生敏感响应的材料,可以对气体浓度、组成等进行检测。
当前,气敏材料的种类繁多,主要包括半导体气敏材料、金属氧化物气敏材料、有机气敏材料等。
半导体气敏材料的制备通常采用溶胶-凝胶法、气相沉积法、离子束溅射法等多种方法,其中,溶胶-凝胶法由于操作简单、成本低廉、制备设备简单等优点,已成为半导体气敏材料制备的首选方法。
溶胶-凝胶法主要是将金属离子或有机物离子与适当的溶剂混合形成胶体,经过凝胶、热处理等工艺制备出气敏材料。
金属氧化物气敏材料的制备主要采用溶胶-凝胶法、物理气相沉积法、化学气相沉积法等方法。
与半导体气敏材料不同,金属氧化物气敏材料的制备通常需要高温煅烧,以提高晶体质量和敏感性。
有机气敏材料的制备主要采用溶剂聚合、原位合成、溶液法等方法,由于有机气敏材料的特殊结构以及溶液制备过程中易于控制,因此在制备过程中需要特别注意溶液粘度、聚合速率等因素。
此外,有机气敏材料的应用范围相对狭窄,多用于检测有机气体或挥发性有机化合物。
从制备过程来看,气敏材料的制备技术难度较大,需要一定的操作技能和实验经验。
另外,制备出来的气敏材料敏感性能也受到多种因素的影响,如晶体结构、纯度、晶界等。
因此,在实际应用中,需要针对具体的检测对象和检测要求进行优化和改进,以提高气敏材料的敏感性和选择性。
气敏材料的气敏性能是用来评价材料对目标气体响应的强弱及可靠性的重要指标之一。
气敏性能包括敏感度、选择性、响应时间、稳定性等指标。
其中,敏感度是评价材料检测目标气体浓度的能力,当目标气体浓度发生一定变化时,敏感度能够反映材料对浓度变化产生的响应。
选择性是评价材料检测目标气体和其他气体的区分能力,即材料对不同气体的响应差异程度。
三种纳米结构三氧化钨的气敏性研究

三种纳米结构三氧化钨的气敏性研究王新刚;郭一凡;田阳;刘丽丽;张怀龙【摘要】Utilizing ammonium metatungstate [(NH4)6W12O40] as raw material, we produced three kinds of nanostructured WO3 under the same reaction conditions by controlling the concentration of citric acid (C6H8O7). The nanostructured WO3 was characterized by XRD, SEM and TEM. Then, three kinds of gas sensors including WO 3 nanorod gas sensors, WO3 nanoplate gas sensors and WO3 nanoplate/nanorods mixing gas sensors were further manufactured. The sensitivity was measured for three kinds of nanostructured WO 3 gas sensors under the condition of acetone, ammonia and formaldehyde gas respectively. Experimental results show that the sensitivities of the three kinds of nanostructured WO3 firstly increase and then decrease with the increase of temperature at gas concentration of 1 000 ×10-6. In contrast, the sensitivity of WO3 nanoplate gas sensors is the highest among the three kinds of nanostructured WO3 for the three kinds of gases in the range of measuring temperature. The opt imum operating temperature of WO3 nanoplate gas sensor is 350 ℃, 300 ℃, 325 ℃, 250 ℃ and its maximum sensitivity is 25.4, 18.52, 30.29, 18.31 in acetone, ammonia and formaldehyde gas, respectively. The sensitivity of the three kinds of nanostructured WO3 firstly increases and then decreases with the increase of temperature in the acetone gas of 50 × 10-6 and the formaldehyde gas of 100×10-6, respectively. The sensitivityof WO3 nanoplate is obviously higher than that of other twonanostructured WO3. At the optimum operating temperature, the acetone and formaldehyde gas with lower concentration can be detected by using nanoplate WO 3 gas sensor.%试验以偏钨酸铵为钨源,采用水热法在相同的反应条件下,通过控制柠檬酸的加入量,合成了三种纳米结构的三氧化钨,并采用XRD、SEM和TEM对合成的WO3粉末进行分析。
铈掺杂WO3纳米材料气敏特性研究
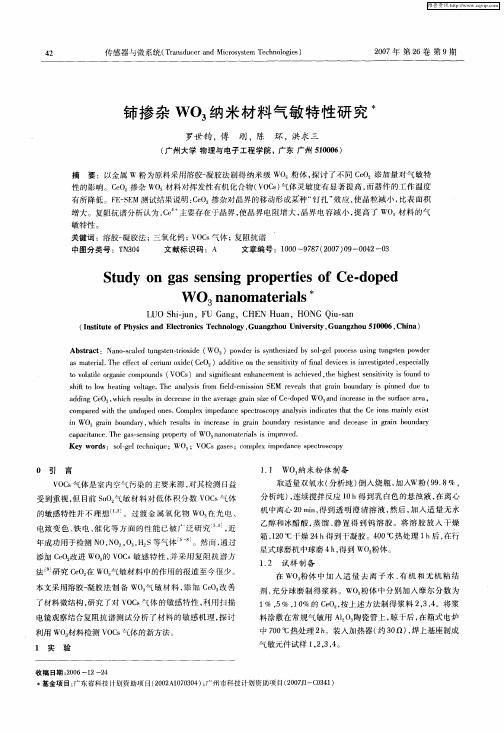
性的影响。C O e 掺杂 wO 材料 对挥发性有机 C) 而器件 的工作温度
(n tueo h s sa dEetoisT c n lg , a gh uU v ri , a gh u5 0 0 , hn ) Is tt f yi n l rnc eh ooy Gu n zo n es y Gu n z o 10 6 C ia i P c c i t A s at aosa dtns ntoie( 3 o dri sn ei db o— l rcs ui ns npw e bt c :N n—cl ugt —i d WO )pw e s yt s e ysl e poes s gt g e o dr r e e rx h z g n u t a ma r 1T ee et f ei xd ( e 2 d iv nt niv y f nl ei s sn et a d epcay s t i .h f c o r m oi C O )adt e es s it o a dv e vsgt ,sei l ea f c u e i o h e t i f c i i i i e l t vl i rai cm on s( O s n i icn n acm n iahee , ehget e sit i fudt o o teognc o p ud V C )ads nf at hn e ets civdt ihs snivy s on al gi e h t i o
s i o lw h ai g v l g . h n lss fo e d e s in S h f t e t ot e T e a ay i r m f l — miso EM e e l t a r i o n a s p n e u o t o n a i r v as h t gan b u d r i i n d d e t y a dn e , h c e u si e r a ei ea e a eg an sz f e d p d W O d i c e s n t e s ra e a e d i g C O2 w ih rs h n d c e s n t v r g r i i o —o e h e C 3 n n r a e i h u fc ra, a c mp r d wi h n o e n s C mp e mp d n e s e to c p n lssi d c t st a h e in il x s o a e t t eu d p d o e . o lx i e a c p c rs o y a ay i n i ae h tt e C o s man y e it h i O3g an b u d r w ih e ut n i c e s n g an b u d r e itn e a d d c a e i an b u d r n W r i o n ay, h c rs l i n r a e i r i o n a r ss c n e e s n g i o n a s y a r y
溶胶-凝胶法制备氧化钨薄膜的研究

溶胶-凝胶法制备氧化钨薄膜的研究刘英;王兵;王速【摘要】H The WO3 thin film was prepared on ceramic substrate by sol-gel method using sodium tungstate as raw material,and then be annealed at 500℃.The structure and morphology of WO3 were characterized by X-ray diffraction(XRD)and scanning electron microscopy(SEM).The results showed that the film surface travels as nano-porous structure.And then,the gas sensing properties of WO3 thin film to NO2, H2 and alcohol were also researched.WO3 showed a better sensitivity to NO2 than to H2 and alcohol.%本文以钨酸盐为原料利用溶胶凝胶法,在陶瓷衬底制备WO3敏感薄膜,于500℃退火。
接着对制备好的薄膜进行X射线衍射与电子显微测试,观察到薄膜表面呈较规则的纳米多孔结构。
接着对NO2、H2、乙醇等气体进行敏感测试,测试证明薄膜对H2、乙醇敏感性较差,对NO2敏感性良好。
【期刊名称】《电子测试》【年(卷),期】2016(000)016【总页数】2页(P160-160,156)【关键词】WO3纳米薄膜;溶胶-凝胶法;气体敏感【作者】刘英;王兵;王速【作者单位】广东宁源科技园发展有限公司,526000;广东宁源科技园发展有限公司,526000;广东宁源科技园发展有限公司,526000【正文语种】中文本文旨在改善溶胶-凝胶法制造工艺,利用后期热处理制造出具有介孔结构的纳米WO3薄膜。
纳米WO3的性质及应用
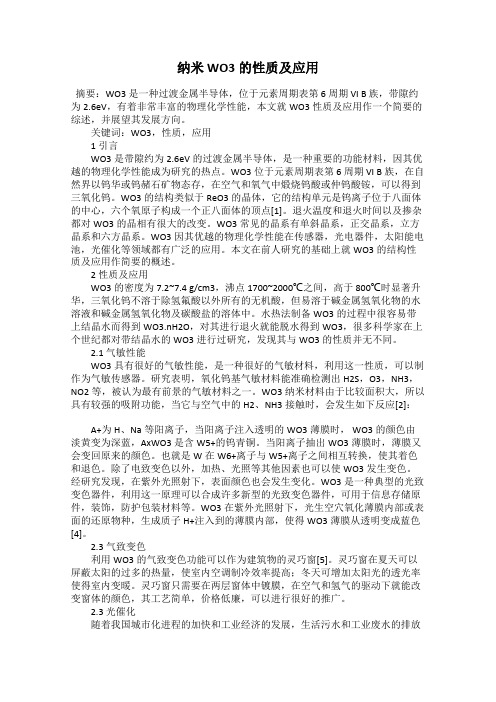
纳米WO3的性质及应用摘要:WO3是一种过渡金属半导体,位于元素周期表第 6 周期 VI B 族,带隙约为2.6eV,有着非常丰富的物理化学性能,本文就WO3性质及应用作一个简要的综述,并展望其发展方向。
关键词:WO3,性质,应用1 引言WO3是带隙约为2.6eV的过渡金属半导体,是一种重要的功能材料,因其优越的物理化学性能成为研究的热点。
WO3位于元素周期表第 6 周期 VI B 族,在自然界以钨华或钨赭石矿物态存,在空气和氧气中煅烧钨酸或仲钨酸铵,可以得到三氧化钨。
WO3的结构类似于ReO3的晶体,它的结构单元是钨离子位于八面体的中心,六个氧原子构成一个正八面体的顶点[1]。
退火温度和退火时间以及掺杂都对WO3的晶相有很大的改变。
WO3常见的晶系有单斜晶系,正交晶系,立方晶系和六方晶系。
WO3因其优越的物理化学性能在传感器,光电器件,太阳能电池,光催化等领域都有广泛的应用。
本文在前人研究的基础上就WO3的结构性质及应用作简要的概述。
2 性质及应用WO3的密度为7.2~7.4 g/cm3,沸点1700~2000℃之间,高于800℃时显著升华,三氧化钨不溶于除氢氟酸以外所有的无机酸,但易溶于碱金属氢氧化物的水溶液和碱金属氢氧化物及碳酸盐的溶体中。
水热法制备WO3的过程中很容易带上结晶水而得到WO3.nH2O,对其进行退火就能脱水得到WO3,很多科学家在上个世纪都对带结晶水的WO3进行过研究,发现其与WO3的性质并无不同。
2.1 气敏性能WO3具有很好的气敏性能,是一种很好的气敏材料,利用这一性质,可以制作为气敏传感器。
研究表明,氧化钨基气敏材料能准确检测出H2S,O3,NH3,NO2等,被认为最有前景的气敏材料之一。
WO3纳米材料由于比较面积大,所以具有较强的吸附功能,当它与空气中的H2、NH3接触时,会发生如下反应[2]:A+为H、Na等阳离子,当阳离子注入透明的WO3薄膜时, WO3的颜色由淡黄变为深蓝,AxWO3是含W5+的钨青铜。
气敏元件的气敏特性影响因素分析
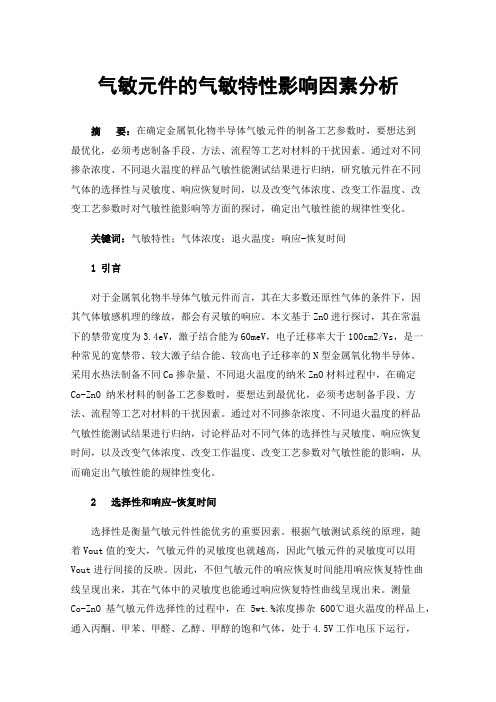
气敏元件的气敏特性影响因素分析摘要:在确定金属氧化物半导体气敏元件的制备工艺参数时,要想达到最优化,必须考虑制备手段、方法、流程等工艺对材料的干扰因素。
通过对不同掺杂浓度、不同退火温度的样品气敏性能测试结果进行归纳,研究敏元件在不同气体的选择性与灵敏度、响应恢复时间,以及改变气体浓度、改变工作温度、改变工艺参数时对气敏性能影响等方面的探讨,确定出气敏性能的规律性变化。
关键词:气敏特性;气体浓度;退火温度;响应-恢复时间1 引言对于金属氧化物半导体气敏元件而言,其在大多数还原性气体的条件下,因其气体敏感机理的缘故,都会有灵敏的响应。
本文基于ZnO进行探讨,其在常温下的禁带宽度为3.4eV,激子结合能为60meV,电子迁移率大于100cm2/Vs,是一种常见的宽禁带、较大激子结合能、较高电子迁移率的N型金属氧化物半导体。
采用水热法制备不同Co掺杂量、不同退火温度的纳米ZnO材料过程中,在确定Co-ZnO 纳米材料的制备工艺参数时,要想达到最优化,必须考虑制备手段、方法、流程等工艺对材料的干扰因素。
通过对不同掺杂浓度、不同退火温度的样品气敏性能测试结果进行归纳,讨论样品对不同气体的选择性与灵敏度、响应恢复时间,以及改变气体浓度、改变工作温度、改变工艺参数对气敏性能的影响,从而确定出气敏性能的规律性变化。
2 选择性和响应-恢复时间选择性是衡量气敏元件性能优劣的重要因素。
根据气敏测试系统的原理,随着Vout值的变大,气敏元件的灵敏度也就越高,因此气敏元件的灵敏度可以用Vout进行间接的反映。
因此,不但气敏元件的响应恢复时间能用响应恢复特性曲线呈现出来,其在气体中的灵敏度也能通过响应恢复特性曲线呈现出来。
测量Co-ZnO基气敏元件选择性的过程中,在5wt.%浓度掺杂600℃退火温度的样品上,通入丙酮、甲苯、甲醛、乙醇、甲醇的饱和气体,处于4.5V工作电压下运行,用来检测不同气体条件下的样品气敏特性,结果如图1所示。
气敏传感器的分类
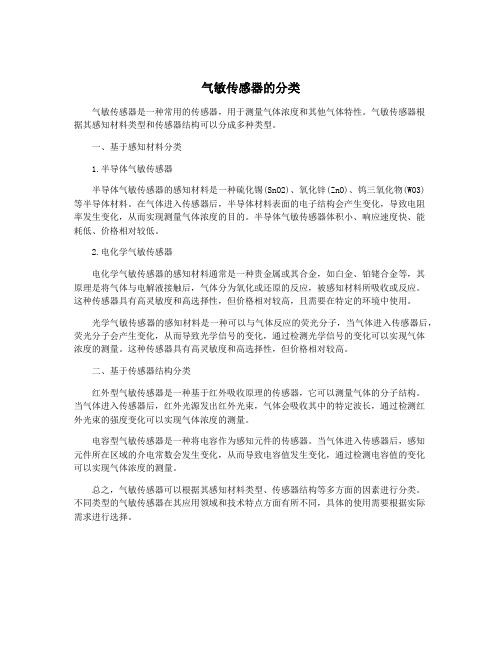
气敏传感器的分类气敏传感器是一种常用的传感器,用于测量气体浓度和其他气体特性。
气敏传感器根据其感知材料类型和传感器结构可以分成多种类型。
一、基于感知材料分类1.半导体气敏传感器半导体气敏传感器的感知材料是一种硫化锡(SnO2)、氧化锌(ZnO)、钨三氧化物(WO3)等半导体材料。
在气体进入传感器后,半导体材料表面的电子结构会产生变化,导致电阻率发生变化,从而实现测量气体浓度的目的。
半导体气敏传感器体积小、响应速度快、能耗低、价格相对较低。
2.电化学气敏传感器电化学气敏传感器的感知材料通常是一种贵金属或其合金,如白金、铂铑合金等,其原理是将气体与电解液接触后,气体分为氧化或还原的反应,被感知材料所吸收或反应。
这种传感器具有高灵敏度和高选择性,但价格相对较高,且需要在特定的环境中使用。
光学气敏传感器的感知材料是一种可以与气体反应的荧光分子,当气体进入传感器后,荧光分子会产生变化,从而导致光学信号的变化,通过检测光学信号的变化可以实现气体浓度的测量。
这种传感器具有高灵敏度和高选择性,但价格相对较高。
二、基于传感器结构分类红外型气敏传感器是一种基于红外吸收原理的传感器,它可以测量气体的分子结构。
当气体进入传感器后,红外光源发出红外光束,气体会吸收其中的特定波长,通过检测红外光束的强度变化可以实现气体浓度的测量。
电容型气敏传感器是一种将电容作为感知元件的传感器。
当气体进入传感器后,感知元件所在区域的介电常数会发生变化,从而导致电容值发生变化,通过检测电容值的变化可以实现气体浓度的测量。
总之,气敏传感器可以根据其感知材料类型、传感器结构等多方面的因素进行分类。
不同类型的气敏传感器在其应用领域和技术特点方面有所不同,具体的使用需要根据实际需求进行选择。
纳米气敏传感器原理
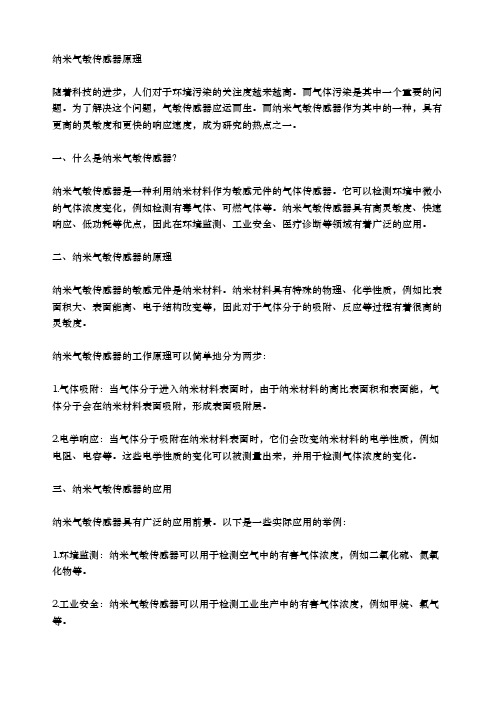
纳米气敏传感器原理随着科技的进步,人们对于环境污染的关注度越来越高。
而气体污染是其中一个重要的问题。
为了解决这个问题,气敏传感器应运而生。
而纳米气敏传感器作为其中的一种,具有更高的灵敏度和更快的响应速度,成为研究的热点之一。
一、什么是纳米气敏传感器?纳米气敏传感器是一种利用纳米材料作为敏感元件的气体传感器。
它可以检测环境中微小的气体浓度变化,例如检测有毒气体、可燃气体等。
纳米气敏传感器具有高灵敏度、快速响应、低功耗等优点,因此在环境监测、工业安全、医疗诊断等领域有着广泛的应用。
二、纳米气敏传感器的原理纳米气敏传感器的敏感元件是纳米材料。
纳米材料具有特殊的物理、化学性质,例如比表面积大、表面能高、电子结构改变等,因此对于气体分子的吸附、反应等过程有着很高的灵敏度。
纳米气敏传感器的工作原理可以简单地分为两步:1.气体吸附:当气体分子进入纳米材料表面时,由于纳米材料的高比表面积和表面能,气体分子会在纳米材料表面吸附,形成表面吸附层。
2.电学响应:当气体分子吸附在纳米材料表面时,它们会改变纳米材料的电学性质,例如电阻、电容等。
这些电学性质的变化可以被测量出来,并用于检测气体浓度的变化。
三、纳米气敏传感器的应用纳米气敏传感器具有广泛的应用前景。
以下是一些实际应用的举例:1.环境监测:纳米气敏传感器可以用于检测空气中的有害气体浓度,例如二氧化硫、氮氧化物等。
2.工业安全:纳米气敏传感器可以用于检测工业生产中的有害气体浓度,例如甲烷、氯气等。
3.医疗诊断:纳米气敏传感器可以用于检测人体呼出气体中的化合物,例如乙醛、甲醛等,从而实现早期疾病的诊断。
四、纳米气敏传感器的发展趋势纳米气敏传感器的发展趋势主要包括以下方面:1.材料优化:随着材料科学的进步,新型纳米材料的开发将会进一步提高纳米气敏传感器的灵敏度和选择性。
2.智能化:纳米气敏传感器将会与智能化技术结合,实现自动化检测、实时监测等功能。
3.微型化:纳米气敏传感器将会越来越小,以适应更多的应用场景。
“气敏性能研究”文件汇整
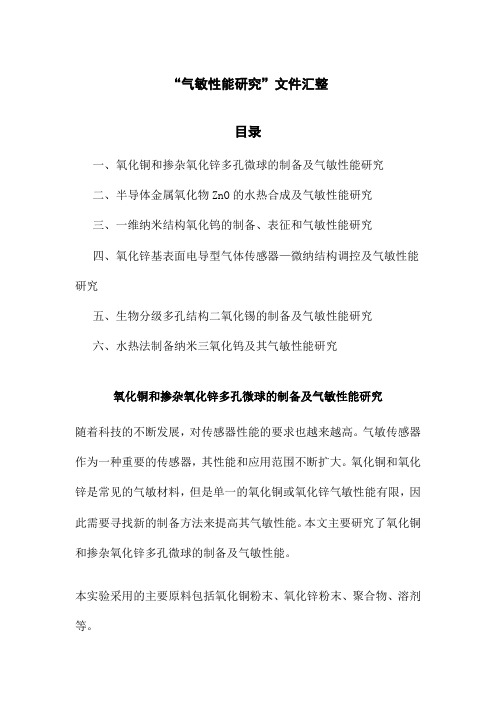
“气敏性能研究”文件汇整目录一、氧化铜和掺杂氧化锌多孔微球的制备及气敏性能研究二、半导体金属氧化物ZnO的水热合成及气敏性能研究三、一维纳米结构氧化钨的制备、表征和气敏性能研究四、氧化锌基表面电导型气体传感器—微纳结构调控及气敏性能研究五、生物分级多孔结构二氧化锡的制备及气敏性能研究六、水热法制备纳米三氧化钨及其气敏性能研究氧化铜和掺杂氧化锌多孔微球的制备及气敏性能研究随着科技的不断发展,对传感器性能的要求也越来越高。
气敏传感器作为一种重要的传感器,其性能和应用范围不断扩大。
氧化铜和氧化锌是常见的气敏材料,但是单一的氧化铜或氧化锌气敏性能有限,因此需要寻找新的制备方法来提高其气敏性能。
本文主要研究了氧化铜和掺杂氧化锌多孔微球的制备及气敏性能。
本实验采用的主要原料包括氧化铜粉末、氧化锌粉末、聚合物、溶剂等。
采用溶胶-凝胶法制备氧化铜和掺杂氧化锌多孔微球。
具体步骤如下:将聚合物、溶剂、氧化铜和氧化锌粉末混合搅拌均匀,形成溶胶;将溶胶置于烘箱中干燥,形成凝胶;将凝胶球破碎、洗涤、干燥,得到多孔微球。
采用恒温恒湿箱进行气敏测试,将制备的多孔微球置于不同浓度的目标气体中,测试其电阻变化。
通过扫描电子显微镜观察制备的多孔微球形貌,发现其具有较好的孔洞结构,孔径分布均匀,有利于气体扩散和吸附。
在测试过程中,发现制备的多孔微球对不同浓度的目标气体具有良好的气敏响应,且响应时间较短。
通过对比纯氧化铜和掺杂氧化锌的多孔微球的气敏性能,发现掺杂氧化锌的多孔微球具有更好的气敏性能。
这可能是因为掺杂氧化锌可以改变材料的电子结构和表面活性,提高气敏响应。
本研究采用溶胶-凝胶法制备了氧化铜和掺杂氧化锌多孔微球,并对其气敏性能进行了研究。
结果表明,制备的多孔微球具有良好的形貌和气敏性能,且掺杂氧化锌可以进一步提高其气敏性能。
这种多孔微球有望应用于气体传感器领域。
半导体金属氧化物ZnO的水热合成及气敏性能研究ZnO是一种常见的宽禁带半导体材料,因其具有高激子束缚能、宽禁带宽度等特点,被广泛应用于各种光电器件和气敏传感器中。
气敏电阻的工作原理及其特性

气敏电阻的工作原理及其特性气敏电阻的工作原理及其特性一、气敏电阻的工作原理及其特性气敏电阻是一种半导体敏感器件,它是利用气体的吸附而使半导体本身的电导率发生变化这一机理来进行检测的。
人们发现某些氧化物半导体材料如SnO2、ZnO、Fe2O3、MgO、NiO、BaTiO3等都具有气敏效应。
常用的主要有接触燃烧式气体传感器、电化学气敏传感器和半导体气敏传感器等。
接触燃烧式气体传感器的检测元件一般为铂金属丝(也可表面涂铂、钯等稀有金属催化层),使用时对铂丝通以电流,保持300℃~400℃的高温,此时若与可燃性气体接触,可燃性气体就会在稀有金属催化层上燃烧,因此,铂丝的温度会上升,铂丝的电阻值也上升;通过测量铂丝的电阻值变化的大小,就知道可燃性气体的浓度。
电化学气敏传感器一般利用液体(或固体、有机凝胶等)电解质,其输出形式可以是气体直接氧化或还原产生的电流,也可以是离子作用于离子电极产生的电动势。
半导体气敏传感器具有灵敏度高、响应快、稳定性好、使用简单的特点,应用极其广泛;半导体气敏元件有N型和P型之分。
N型在检测时阻值随气体浓度的增大而减小;P型阻值随气体浓度的增大而增大。
象SnO2金属氧化物半导体气敏材料,属于N型半导体,在200~300℃温度它吸附空气中的氧,形成氧的负离子吸附,使半导体中的电子密度减少,从而使其电阻值增加。
当遇到有能供给电子的可燃气体(如CO等)时,原来吸附的氧脱附,而由可燃气体以正离子状态吸附在金属氧化物半导体表面;氧脱附放出电子,可燃行气体以正离子状态吸附也要放出电子,从而使氧化物半导体导带电子密度增加,电阻值下降。
可燃性气体不存在了,金属氧化物半导体又会自动恢复氧的负离子吸附,使电阻值升高到初始状态。
这就是半导体气敏元件检测可燃气体的基本原理。
目前国产的气敏元件有2种。
一种是直热式,加热丝和测量电极一同烧结在金属氧化物半导体管芯内;另一种是旁热式,这种气敏元件以陶瓷管为基底,管内穿加热丝,管外侧有两个测量极,测量极之间为金属氧化物气敏材料,经高温烧结而成。
《纳米碳材料的制备及其用于气态硫化物脱除的性能研究》

《纳米碳材料的制备及其用于气态硫化物脱除的性能研究》一、引言随着工业化和城市化的快速发展,大气污染问题日益突出,其中气态硫化物的排放是导致空气质量恶化的主要因素之一。
因此,研究高效、环保的气态硫化物脱除技术对于环境保护具有重要意义。
纳米碳材料因其独特的物理化学性质,在气体吸附、催化等领域具有广泛应用。
本文旨在研究纳米碳材料的制备方法及其在气态硫化物脱除中的应用性能。
二、纳米碳材料的制备1. 制备方法纳米碳材料的制备方法主要包括化学气相沉积法、模板法、溶胶凝胶法等。
本文采用化学气相沉积法,以甲烷为碳源,通过高温裂解制备纳米碳材料。
2. 制备过程(1)将甲烷与惰性气体混合,形成反应气体;(2)将反应气体通入高温反应炉中,进行裂解反应;(3)反应后得到纳米碳材料,通过收集、提纯得到纯净的纳米碳材料。
三、纳米碳材料用于气态硫化物脱除的性能研究1. 实验原理纳米碳材料具有较高的比表面积和孔隙率,能够吸附气态硫化物。
同时,纳米碳材料还具有较好的催化性能,能够促进硫化物的氧化反应。
因此,纳米碳材料在气态硫化物脱除中具有较好的应用潜力。
2. 实验方法(1)制备不同粒径的纳米碳材料;(2)将纳米碳材料装填在固定床反应器中;(3)通入含有气态硫化物的气体,进行吸附和催化反应;(4)检测反应前后气体中硫化物的浓度,计算脱除效率。
3. 实验结果及分析(1)粒径对脱除效率的影响:随着纳米碳材料粒径的减小,比表面积和孔隙率增大,脱除效率逐渐提高。
当粒径达到一定值时,脱除效率达到最大值。
(2)温度对脱除效率的影响:随着温度的升高,气态硫化物的脱除效率先增加后降低。
在一定的温度范围内,纳米碳材料的吸附和催化性能达到最佳状态。
(3)与其他材料的对比:将纳米碳材料与其他吸附剂和催化剂进行对比,发现纳米碳材料在气态硫化物脱除方面具有较高的效率和较好的稳定性。
四、结论本文通过化学气相沉积法制备了纳米碳材料,并研究了其在气态硫化物脱除中的应用性能。
硫化氢气体传感器的研究进展

硫化氢气体传感器的研究进展吴丽倩;宋红杰;吕弋【摘要】硫化氢(H2S)是一种具有臭鸡蛋气味的腐蚀性气体,是广泛存在的环境污染物之一.H2S为剧毒性气体,且易挥发,严重损伤人的呼吸系统,甚至神经系统和心脏器官.因此,实现H2S气体的快速、高灵敏及实时检测对于工业生产、公共安全、环境监测和医疗健康等领域具有十分重要的意义.该文对近5年来电量型和光学型两类H2S气体传感器在传感技术和传感材料上取得的重要进展进行了综述,并对这两类传感器所面临的问题和未来发展趋势进行了探讨.【期刊名称】《分析测试学报》【年(卷),期】2018(037)010【总页数】7页(P1192-1198)【关键词】硫化氢;电量型气体传感器;光学气体传感器;综述【作者】吴丽倩;宋红杰;吕弋【作者单位】四川大学化学学院,四川成都610064;四川大学化学学院,四川成都610064;四川大学化学学院,四川成都610064;四川大学分析测试中心,四川成都610064【正文语种】中文【中图分类】O659.31;TP212.2硫化氢(H2S)是一种无色、有刺激性气味的气体,通常产生于煤、石油、天然气和温泉中。
另外,有机物分解、冶炼、废水处理、填埋、制革等过程也是H2S的重要产生源。
H2S作为一种大气污染物,严重影响人类的生活质量,已被职业安全与健康标准列为有毒且高危险的化学品。
较低浓度的H2S气体即可损伤人的呼吸系统及神经系统[1],长期暴露或高浓度接触会造成头晕、呕吐甚至死亡[2-3]。
而H2S气体的可燃性能导致爆炸,给公共安全带来了巨大的威胁。
因此,针对H2S气体的检测一直是科研工作者研究的热点课题。
目前,H2S的常用检测方法有碘量法、亚甲蓝分光光度法[4]、气相色谱法[5-6]、液相色谱法[7]等。
其中,气相色谱是使用最多且精确度最高的方法。
然而,此方法操作步骤繁琐,且大气环境短时间内变化剧烈,因此无法实现大气环境的实时同步监测。
近年来,气体传感因在实时监测大气污染物方面具有独特优势而备受关注,而气体传感器是将气体浓度转换为相应的可输出信号的器件,在工业生产控制、家庭安全报警、食品工业、临床诊断、环境保护和国土安全等方面有着重要的应用。
WO3气敏传感材料及器件的研究进展
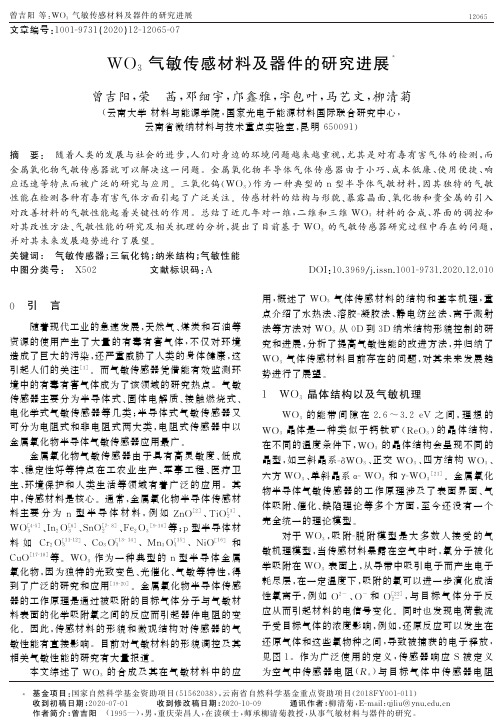
文章编号:1001-9731(2020)12-12065-07WO3气敏传感材料及器件的研究进展*曾吉阳,荣茜,邓细宇,邝鑫雅,字包叶,马艺文,柳清菊(云南大学材料与能源学院,国家光电子能源材料国际联合研究中心,云南省微纳材料与技术重点实验室,昆明650091)摘要:随着人类的发展与社会的进步,人们对身边的环境问题越来越重视,尤其是对有毒有害气体的检测,而金属氧化物气敏传感器就可以解决这一问题㊂金属氧化物半导体气体传感器由于小巧㊁成本低廉㊁使用便捷㊁响应迅速等特点而被广泛的研究与应用㊂三氧化钨(WO3)作为一种典型的n型半导体气敏材料,因其独特的气敏性能在检测各种有毒有害气体方面引起了广泛关注㊂传感材料的结构与形貌㊁暴露晶面㊁氧化物和贵金属的引入对改善材料的气敏性能起着关键性的作用㊂总结了近几年对一维,二维和三维WO3材料的合成㊁界面的调控和对其改性方法㊁气敏性能的研究及相关机理的分析,提出了目前基于WO3的气敏传感器研究过程中存在的问题,并对其未来发展趋势进行了展望㊂关键词:气敏传感器;三氧化钨;纳米结构;气敏性能中图分类号: X502文献标识码:A D O I:10.3969/j.i s s n.1001-9731.2020.12.0100引言随着现代工业的急速发展,天然气㊁煤炭和石油等资源的使用产生了大量的有毒有害气体,不仅对环境造成了巨大的污染,还严重威胁了人类的身体健康,这引起人们的关注[1]㊂而气敏传感器凭借能有效监测环境中的有毒有害气体成为了该领域的研究热点㊂气敏传感器主要分为半导体式㊁固体电解质㊁接触燃烧式㊁电化学式气敏传感器等几类;半导体式气敏传感器又可分为电阻式和非电阻式两大类,电阻式传感器中以金属氧化物半导体气敏传感器应用最广㊂金属氧化物气敏传感器由于具有高灵敏度㊁低成本㊁稳定性好等特点在工农业生产㊁军事工程㊁医疗卫生㊁环境保护和人类生活等领域有着广泛的应用㊂其中,传感材料是核心㊂通常,金属氧化物半导体传感材料主要分为n型半导体材料,例如Z n O[2]㊁T i O[3]2㊁WO[4-5]3㊁I n2O[6]3㊁S n O[7-8]2㊁F e2O3[9-10]等;p型半导体材料如C r2O[11-12]3㊁C o3O[13-14]4㊁M n3O[15]4㊁N i O[16]和C u O[17-18]等㊂WO3作为一种典型的n型半导体金属氧化物,因为独特的光致变色㊁光催化㊁气敏等特性,得到了广泛的研究和应用[19-20]㊂金属氧化物半导体传感器的工作原理是通过被吸附的目标气体分子与气敏材料表面的化学吸附氧之间的反应而引起器件电阻的变化㊂因此,传感材料的形貌和微观结构对传感器的气敏性能有直接影响㊂目前对气敏材料的形貌调控及其相关气敏性能的研究有大量报道㊂本文综述了WO3的合成及其在气敏材料中的应用,概述了WO3气体传感材料的结构和基本机理,重点介绍了水热法㊁溶胶-凝胶法㊁静电纺丝法㊁离子溅射法等方法对WO3从0D到3D纳米结构形貌控制的研究和进展,分析了提高气敏性能的改进方法,并归纳了WO3气体传感材料目前存在的问题,对其未来发展趋势进行了展望㊂1 WO3晶体结构以及气敏机理WO3的能带间隙在2.6~3.2e V之间,理想的WO3晶体是一种类似于钙钛矿(R e O3)的晶体结构,在不同的温度条件下,WO3的晶体结构会呈现不同的晶型,如三斜晶系-δWO3㊁正交WO3㊁四方结构WO3㊁六方WO3㊁单斜晶系α-WO3和γ-WO3[21]㊂金属氧化物半导体气敏传感器的工作原理涉及了表面界面㊁气体吸附㊁催化㊁缺陷理论等多个方面,至今还没有一个完全统一的理论模型㊂对于WO3,吸附-脱附模型是大多数人接受的气敏机理模型,当传感材料暴露在空气中时,氧分子被化学吸附在WO3表面上,从导带中吸引电子而产生电子耗尽层,在一定温度下,吸附的氧可以进一步演化成活性氧离子,例如O2-㊁O-和O[22]2-,与目标气体分子反应从而引起材料的电信号变化㊂同时也发现电荷载流子受目标气体的浓度影响,例如,还原反应可以发生在还原气体和这些氧物种之间,导致被捕获的电子释放,见图1㊂作为广泛使用的定义,传感器响应S被定义为空气中传感器电阻(R a)与目标气体中传感器电阻56021曾吉阳等:WO3气敏传感材料及器件的研究进展*基金项目:国家自然科学基金资助项目(51562038),云南省自然科学基金重点资助项目(2018F Y001-011)收到初稿日期:2020-07-01收到修改稿日期:2020-10-09通讯作者:柳清菊,E-m a i l:q j l i u@y n u.e d u.c n 作者简介:曾吉阳(1995 ),男,重庆荣昌人,在读硕士,师承柳清菊教授,从事气敏材料与器件的研究㊂(R g )的比值(R a /R g )㊂有时,响应S 被定义为相对电阻变化,即S =(R a -R g )/R g ㊂响应和恢复时间为传感器在吸附和解吸过程中完成总电阻变化的90%所需的时间㊂此外,其他参数如最佳工作温度(T )㊁选择性㊁检测极限㊁重复性和长期稳定性对于综合评估传感特性也很重要㊂图1 O 2和H 2S 分子在I n 2O 3@WO 3纳米层系结构界面的吸附和反应过程示意图[23]F i g 1As c h e m a t i cd e m o n s t r a t i o no f t h ea d s o r pt i o n a n d r e a c t i o n p r o c e s s o fO 2a n dH 2Sm o l e c u l e s a tt h ei n t e r f a c eo ft h e h i e r a r c h i c a lI n 2O 3@WO 3na n o s t r u c t u r e [23]由上述机理可知,金属氧化物气敏传感器的传感性能与其表面的氧空位有直接关系,形成氧空位越多,表面吸附氧物种越多,气敏性能越高㊂而晶面上原子的不同排列方式和悬挂键数量是不同晶面氧空位形成差异的关键㊂近年来,许多研究证实了在具有高能量暴露面的可控形貌显示出很好的传感性能㊂H a n [24]和他的同事报道过使用酒石酸来调节水热法合成的三羧酸WO 3纳米薄片中(001)㊁(100)和(010)的比例,研究表明暴露较多(010)面的样品对1-丁胺表现出更好的气敏特性㊂J i a 等[25]采用水热法合成暴露(100)和(002)晶面的WO 3纳米棒,结果表明,暴露(002)晶面的WO 3对丙酮的气敏性能比暴露(100)晶面的更好㊂T i a n 等[26]以末端为WO 的六角状WO 3的(001)面为基准,揭示了氧密度主导的气敏机理㊂研究发现,高氧密度有利于WO 3对CO 和H 2等还原气体的气敏性能㊂因此,晶面原子的不同排列方式对材料表面氧空位的形成产生影响,从而影响气敏性能㊂2 WO 3气敏传感器的研究进展2.1 零维结构WO 3气敏材料零维结构的材料主要为纳米球㊁纳米颗粒和量子点㊂关于WO 3纳米粒子气敏传感的报道并不是很多,虽然颗粒纳米化后的比表面积有所提高,但是不足的是纳米化后造成的大的界面阻碍了电子迁移,因此经常气敏性能不理想[27]㊂然而通过掺杂或者贵金属改性,可以提高其气敏性能㊂利用贵金属对WO 3进行改性,主要是利用贵金属的敏化及催化作用,敏化包括化学敏化和电子敏化㊂化学敏化主要是将氧分子解离成化学吸附氧,通过之前气敏机理分析可知,化学吸附氧数量的增加将会提高材料的气敏性能㊂电子敏化主要是让贵金属和金属氧化物之间形成肖特基接触,提高电子的移动速率,进而提高材料的气敏性能㊂催化则是催化分子氧的离解,以促进氧分子转化为WO 3表面上的活性氧物种㊂同时贵金属与WO 3表面发生相互作用,导致WO 3费米能级的变化,也有利于气敏性能的提高㊂L i 等[28]通过简单的水热合成法向WO 3纳米颗粒中掺杂入A u 原子,提高了WO 3纳米颗粒对二甲苯气体的响应,在225ħ的工作温度下有效检测浓度为5ˑ10-6,响应值为26.5,检测极限能到200p pb ㊂A u 的掺入催化分子氧的解离,使化学吸附氧的数量增加,并且可以降低二甲苯脱氢的活化能,使其更容易分解,导致更高的响应值㊂L i 等[29]采用了硫酸酸化N a 2WO 4制备了WO 3纳米粒子,并利用基于电子吸附机理的改性浸渍法对纳米粒子表面进行了R u 催化改性,得到了平均粒径为28n m 的R u 负载的WO 3纳米颗粒,对人体呼出气体中的丙酮进行检测,在300ħ下检测到1.5ˑ10-6浓度的丙酮气体,响应值为6.8,是纯的WO 3纳米颗粒的5倍,气敏性能得到了显著提高,这是由于R u 负载在WO 3表面是氧化态,产生电子敏化效应,提高电子转移速率㊂2.2 一维结构WO 3气敏材料在各种WO 3纳米结构中,一维WO 3结构例如纳米棒㊁空纳米管㊁多孔纳米线和纳米纤维因具有较高的比表面积,同时保持良好的化学和热稳定性,这使其电学性质对表面化学吸附非常敏感,使得有效面积和活性位点比其他结构高,已被证明可有效提高气敏性能㊂Q i n 等[30]通过溶剂热法合成了具有准取向的W 18O 49纳米线,在450ħ的退火温度下所得到的产物结构高度疏松,交联孔使气体的扩散更加容易,制成的气敏传感器对N O 2气体展现出高的灵敏度和较快的响应速度㊂单纯的WO 3无法满足较高的气敏要求,通过掺杂其他金属氧化物构建半导体异质结可以有效提高材料的气敏性能㊂WO 3为n 型半导体,通过掺杂p 型半导体或者n 型半导体,可以构成p -n 异质结[31]或者n -n异质结[32],促进电子迁移,提高气敏性能㊂S e u n g b o k C h o i 等[33]通过在氧化气氛中热蒸发WO 3粉末,合成了由C r 2O 3纳米颗粒修饰的一维WO 3纳米棒,形成了C r 2O 3-WO 3的p -n 结,对2ˑ10-4乙醇气体的响应值为5.58,是纯的WO 3纳米棒的4.4倍,C r 2O 3进入WO 3的晶格中,形成晶格缺陷,增加乙醇气体的附着点,导致气敏性能的提高㊂Q i n 等[34]通过诱导溅射法制备了一种高度有序排列的C u O -WO 3核壳结构的纳米棒状材料,使工作温度降至室温,并且对N O 2有着660212020年第12期(51)卷良好的响应与选择性,这些结果与核-壳界面异质结势垒和导电载流子积累通道的独特排列有关如图2所示㊂F e n g等[35]则采用P t@M I L-101和静电纺丝技术设计了P t-C r2O3-W O3复合纳米纤维,P t纳米颗粒的尺寸为3n m,对二甲苯表现出优异的响应,对5ˑ10-6二甲苯的响应值为75.3㊂增强的气敏性能归因于C r2O3-W O3异质结㊁C r2O3的催化活性㊁多孔结构㊁P t纳米粒子的电子和化学敏化㊂多孔纳米棒和纳米管等分级结构在气敏领域的应用也受到了广泛关注㊂W o n-T a e K o o等[36]采用逐层自组装的方法,在聚合纳米纤维模板上采用静电纺丝方法制备了多孔W O3纳米管,在高湿度(90%)的环境下,纯的WO3纳米管对5ˑ10-6N O的响应值为63.59,这是因为高湿度大气中的水分子分解成羟基,并将电子传递给W O3㊂D i n g等[37]则以棉纤维作为模板,通过简单的渗透和煅烧工艺合成了新型掺杂碳的W O3微管,碳的引入将W O3的带隙从2.45e V降到了2.12e V,W O3纳米管是由约40n m的纳米粒子组成,其内部存在大量的中空和大孔,使其拥有更大的比表面积,在90ħ的工作温度下对0.5ˑ10-6的甲苯响应值为40,检测极限为50p p b㊂S u n g h o o nP a r k等[38]则使用热蒸发技术合成了W O3纳米棒,并用离子溅射方法在W O3纳米棒表面负载了P t颗粒,P t颗粒负载使含氧物质的溢出㊁气体化学吸附和解离增强,改善了W O3纳米材料对C O和N O2气体的响应㊂图2 W18O49/C u O核壳纳米棒阵列气敏机理示意图:(a)N O2吸附示意图;(b)能带平衡前图;(c)能带平衡后图[34]F i g2S c h e m a t i c i l l u s t r a t i o no f t h e g a s-s e n s i n g m e c h a n i s mf o r t h eW18O49/C u Oc o r e-s h e l l n a n o r o d s a r r a y:(a)N O2a d s o r p t i o nd i a g r a m;(b,c)e n g e r y b a n dd i a g r a m so f t h e W18O49/C u Oc o r e-s h e l l n a n o r o d sb e f o r ea n d a f t e r e q u i l ib r i u m[34]2.3二维结构WO3气敏材料典型的二维WO3结构如薄膜㊁纳米片或纳米板,由于其高的比表面积㊁表面活性㊁表面极化和丰富的氧空位等而使其具有良好的气敏性能㊂S.S.S h e n d a g e 等[39]使用水热法合成了WO3薄膜材料,薄膜厚为90 ~150n m,在100ħ的工作温度下,对5ˑ10-6的N O2气体的响应为10㊂A b d e l h a m i dB o u d i b a等[40]使用水热法在180ħ的环境下反应48h合成WO3纳米板,在200~300ħ的工作温度与高湿度(50%)的环境下对S O2气体有着良好的响应,最低检测浓度为1ˑ10-6㊂T a n等[41]在陶瓷衬底上采用电子束蒸发沉积WO3薄膜,然后通过原子层沉积(A L D)在WO3薄膜表面沉积R h纳米颗粒㊂当R h的A L D沉积周期为20时,样品表现出最好的选择性,与纯的WO3相比,选择性提高了约110%,在350ħ的工作温度下,对5ˑ10-6的C H4有着63.1的响应值,且最低检测浓度为0.1ˑ10-6,这可能是由于R h纳米粒子的表面催化作用㊂T a r oU e d a等[5]通过硝酸酸化钨酸钠制备WO3薄膜,再将R u掺入WO3中,R u的加入不仅使WO3对甲硫醇的最佳工作温度从175ħ降至125ħ,还提高了其对甲硫醇的催化活性,可以将甲硫醇降解为C O2,S O2和H2O㊂L i等[23]在WO3纳米片表面进行插入和拓扑化学转化,通过微波辅助在WO3纳米片上合成了分级I n2O3纳米复合材料(I n2O3@WO3),尺寸范围为12~20n m的I n2O3纳米粒子均匀固定在WO3纳米片的表面上,I n2O3@WO3样品在150ħ下对1ˑ10-576021曾吉阳等:WO3气敏传感材料及器件的研究进展H2S的响应高达143,是WO3纳米片的4倍,是纯I n2O3纳米晶体的13倍㊂石墨烯是一种新型二维材料,它由碳原子以s p2杂化轨道组成六角型呈蜂巢晶格,自发现以来,因其优异的力学㊁电学㊁光学等特性,在众多科研领域得到应用㊂石墨烯具有热稳定性好㊁比表面大的特点,表面丰富的缺陷以及官能团可提供大量的化学活性位点,此外,较大的比表面积有利于WO3等其他传感材料分布均匀,通过协调效应增强传感性能㊂石墨烯与WO3复合,可以有效提高WO3的气敏性能㊂N o v i k o v等[42]合成了基于外延石墨烯的气体传感器并对其进行了优化,并通过进一步的退火显著改善了气敏性能,对N O2气体的响应比未退火石墨烯传感器的响应值高十倍,并且检测下限为0.6p p b㊂性能的提高是由于制备的氧化石墨烯-WO3复合纳米纤维具有特殊的多孔结构,并且氧化石墨烯纳米片与WO3纳米颗粒之间形成欧姆接触,有效改善了材料的比表面积和气体吸附能力㊂近些年石墨氮化碳(g-C3N4)因其优异的性能以及大的比表面积,吸引了众多关注㊂S.V i j a y a k u m a r 等[43]采用微波辐射制备了WO3-石墨氮化碳复合材料,WO3纳米颗粒分散于g-C3N4表面,制备成聚甲基丙烯酸甲酯光纤包层的光纤气体传感器,对乙醇有较好的选择性和灵敏度㊂2.4三维结构WO3气敏材料三维WO3是由纳米粒子㊁纳米片形成分级结构,典型的形状有微球㊁纳米花状㊁海胆状结构㊁介孔结构等不规则结构㊂由于独特的形貌结构,因此具有较大的比表面积㊁丰富的孔隙率,使其对各种气体有较高的气敏性能㊂因此,与其他维度的WO3传感材料相比, 3D分级多孔结构被认为是更有发展潜力的气体传感材料㊂W a n g等[44]通过钨酸钠酸化方法合成了由多个不规则的纳米片组成的纳米花状结构,由这些层片结构组成的传感器在90ħ条件下检测N O2气体,气体浓度为2p p b时,灵敏度达到12.8㊂J i e等[45]通过溶胶凝胶法地制备了石墨烯包覆的WO3纳米球状结构,通过测试气敏性能发现,G R包裹的WO3纳米微球复合材料表现出p型气体敏感行为,并且G R-WO3传感器对N O2气体的响应在室温条件下,随着气体浓度的增加而线性增加㊂Z h a o等[46]合成了大孔径(13n m)㊁高比表面积(128m2/g)的具有面心立方(F C C)有序介孔结构的WO3/P t纳米复合材料,如图3所示㊂尺寸为4n m的铂纳米颗粒均匀分布在孔中,通过化学和电子手段使WO3基质敏化以用于C O检测㊂图3 P E O-b-P S模板合成有序中孔WO3/P t过程[46]F i g3T h e s y n t h e s i s p r o c e s s o f o r d e r e dm e s o p o r o u sWO3/P tw i t hP E O-b-P S t e m p l a t e[46]模板法是利用已有的内在特征来调控所需纳米材料的形貌㊂根据模板的类型,可以得到空心球㊁空心六棱柱和介孔等不同形貌的WO3㊂碳球作为模板被广泛用于制备高质量的WO3空心球气敏材料,根据模板的形成分为两步法[47]和一步法[48]㊂此外,有团队还利用莲花花粉等生物模板制备了具有高孔双壳结构的WO3微球,完美地继承了模板的结构特征㊂在200ħ条件下,WO3微球对1ˑ10-4N O的灵敏度为46.2,响应和恢复时间分别为62和223s[49]㊂W u等[50]使用硅基作为硬模板合成了介孔P d负载的氢气传感器㊂P d的加入提高了WO3对H2的响应值和缩短了响应与恢复时间㊂Z h u等[51]合成了一种WO3-Z n O中空纳米球状的混合物,由42n m的颗粒组合而成,对丙酮气体有良好的选择性和响应值㊂柔性气体传感因在可穿戴和便携式电子产品㊁射频识别标签和监测易腐货物的智能包装中的潜在应用,引起了科学界的高度兴趣[52]㊂而聚吡咯(P P y)㊁聚苯胺(P A N I)和聚噻吩(P T h)等导电聚合物具有优异电学和力学性能㊂WO3通常与导电聚合物复合制备传感膜,在柔性器件和室温检测方面具有突出优势㊂860212020年第12期(51)卷H u a n g等[53]在柔性基板上使用电子印刷技术制备了WO3-P E D O T/P P SN O2气体传感器,与纯WO3传感器相比,传感性能的增强得益于P E D O T:P S S提供的导电通道㊁WO3-P E D O T:P S S界面上的形成的异质结㊂L i等[54]采用简易的原位化学氧化聚合方法合成了花状WO3纳米复合材料,并将其负载在柔性聚对苯二甲酸乙二醇酯(P A N I)基底上,制备了室温下工作的N H3传感器,与纯聚苯胺有机气敏传感器相比较,对N H3的响应值高6倍㊂H e等[31]通过两步水热合成法制作了表面由C u O修饰的WO3纳米花状的气敏传感器,在80ħ的工作温度下对H2S有着良好的响应㊂S u n等[55]在柔性P E T衬底上制备了用于智能三乙胺(T E A)传感器的P P y@WO3杂化物,在室温下对T E A具有高的灵敏度㊁良好的选择性,归因于WO3和P P y间的互补效应和界面形成了p-n异质结㊂3结语重点介绍了WO3气敏传感材料的研究现状及进展,综述了不同维度㊁不同尺寸㊁不同形貌的WO3气敏传感材料,以及贵金属和氧化物对其界面的修饰和改性㊂零维WO3纳米材料的比表面积与活性位点相对其他维度的更低,制备过程中更容易团聚,使颗粒粗化;一维WO3如纳米线㊁纳米棒等则具有较高的比表面积,使得有效面积和有效活性位点比其他结构高,使得有效活性位点比其他结构高,同时保持良好的化学和热稳定性,使其电学性质对表面化学吸附非常敏感;二维WO3如纳米片㊁纳米薄膜等则拥有可调制的表面活性㊁表面极化和富氧空位等特性,能够提供更多的吸附位点;三维WO3具有高的孔隙率,各种独特的形貌,如核-壳结构㊁介孔分级结构等,为氧离子和吸附气体能够提供更多的通道与孔洞㊂虽然WO3气敏材料性能的研究取得了一定的成果,但是还存在以下不足:对目标气体的选择性㊁灵敏度㊁稳定性不理想;工作温度一般在25~400ħ之间,高的工作温度既不利于传感器的长期稳定性,也增加了使用能耗㊂由于这些不足,影响了WO3气敏传感材料的发展,后续研发重点及发展趋势如下:(1)进一步从材料结构㊁能带结构㊁导电性能等方面对WO3气敏传感材料进行调控,有效降低工作温度,提高对目标气体的灵敏度和选择性㊂(2)抗湿性是目前WO3气敏传感材料在实际应用中的首要工作,高湿度环境下的气敏检测越发的重要,研制出能在潮湿的环境下具有高灵敏度㊁高选择性和高稳定性的WO3材料是一个重要的方向㊂(3)WO3气敏传感材料气敏性能变化的原因还需要从微观角度进一步分析㊂在对实验结果进行合理解释的基础上,依据材料固有性质及特殊的表面性质建立物理模型,深入分析并阐明其气敏机理㊂参考文献:[1] Z h a n g J,L i uX H,N e r i G,e t a l.N a n o s t r u c t u r e dm a t e r i-a l s f o r r o o m-t e m p e r a t u r e g a s s e n s o r s[J].A d v a n c e d M a-t e r i a l s,2016,28(5):795-831.[2] B h a t iVS,H o j a m b e r d i e v M,K u m a rM.E n h a n c e ds e n s-i n gp e r f o r m a n c eo fZ n O n a n o s t r u c t u r e s-b a s e d g a ss e n-s o r s:a r e v i e w[J].E n e r g y R e p o r t s,2020,6:46-62.[3] S e e k a e w Y,W i s i t s o r a a tA,P h o k h a r a t k u l D,e t a l.R o o mt e m p e r a t u r e t o l u e n e g a s s e n s o rb a s e do nT i O2n a n o p a r t i-c l e sde c o r a t e d3D g r a p h e n e-c a r b o n n a n o t u b en a n o s t r u c-t u r e s[J].S e n s o r sa n d A c t u a t o r s B:C h e m i c a l,2019, 279:69-78.[4] K u l k a r n iSB,N a v a l eY H,N a v a l eS T,e ta l.H y b r i dp o l y a n i l i n e-WO3f l e x i b l e s e n s o r:a r o o m t e m p e r a t u r ec o m p e t e n c e t o w a rd sN H3g a s[J].Se n s o r s a n dA c t u a t o r sB:C h e m i c a l,2019,288:279-288.[5]U e d a T,M a e d a T,H u a n g Z,e ta l.E n h a n c e m e n to fm e t h y l m e r c a p t a ns e n s i n g r e s p o n s e o fWO3s e m i c o n d u c t o rg a s s e n s o r sb yg a s r e a c t i v i t y a n d g a s d i f f u s i v i t y[J].S e n-s o r s a n dA c t u a t o r sB:C h e m i c a l,2018,273:826-833.[6]W a n g Y C,S u nZS,W a n g SZ,e ta l.S u b-p p m a c e t i ca c i d g a s s e n s o rb a s e do n I n2O3n a n o f i b e r s[J].J o u r n a l o fM a t e r i a l sS c i e n c e,2019,54(22):14055-14063.[7] H o n g PP,M a n hH C,T o a nN V,e t a l.O n e-s t e p f a b r i-c a t i o no f S n O2p o r o u sn a n o f i b e r g a s s e n s o r s f o r s u b-p p mH2Sd e t e c t i o n[J].S e n s o r sa n d A c t u a t o r s A:P h y s i c a l, 2020,303:111722(1-11).[8] Z h a n g R,X uZ,Z h o uT,e t a l.I m p r o v e m e n t o f g a s s e n s-i n gp e r f o r m a n c e f o r t i nd i o x i d es e n s o r t h r o u g hc o n s t r u c-t i o no fn a n o s t r u c t u r e s[J].J o u r n a l o fC o l l o i da n dI n t e r-f a c eS c i e n c e,2019,557:673-682.[9] T o u b aS,K i m i a g a r S.E n h a n c e m e n t o f s e n s i t i v i t y a n d s e-l e c t i v i t y o f a l p h a-F e2O3n a n o r o d g a s s e n s o r s b y Z n On a n-o p a r t i c l e s d e c o r a t i o n[J].M a t e r i a l sS c i e n c ei nS e m i c o n-d u c t o rP r o ce s s i n g,2019,102:104603(1-8).[10] W a n g X,W a n g TK,S i GK,e t a l.O x y g e n v a c a n c y d e-f e c t se ng i n e e r i n g o n C e-d o p e da l ph a-F e2O3g a ss e n s o rf o r r e d u c i ng g a s e s[J].S e n s o r s a n dA c t u a t o r s B:Ch e m i-c a l,2020,302:127165(1-8).[11] C h o i S,B o n y a n iM,S u nGJ,e t a l.C r2O3n a n o p a r t i c l e-f u n c t i o n a l i z e dWO3n a n o r o d s f o r e t h a n o lg a s s e n s o r s[J].A p p l i e dS u r f a c eS c i e n c e,2018,432:241-249.[12] W a n g T Y,L iY Y,L iT T,e t a l.E n h a n c e dN O x g a ss e n s i n gp r o p e r t i e s o f C r2O3f i l m m o d i f i e d o r d e r e d p o r o u sZ n O g a ss e n s o r s[J].S o l i d S t a t eI o n i c s,2018,326:173-182.[13] T i a n M,M i a oJ,C h e n g P,e t a l.L a y e r-b y-l a y e rn a n o-c o m p o s i t e sc o n s i s t i n g o fC o3O4a n dr ed u ce d g r a p h e n e(r G O)n a n o s h e e t sf o rh i g hs e l e c t i v i t y e t h a n o l g a ss e n-s o r s[J].A p p l i e dS u r f a c eS c i e n c e,2019,479:601-607.[14] Z h a n g X,W a n g J,X u a nL,e t a l.N o v e lC o3O4n a n o-c r y s t a l l i n e c h a i nm a t e r i a l a s ah i g h p e r f o r m a n c e g a s s e n-s o ra tr o o m t e m p e r a t u r e[J].J o u r n a lo f A l l o y sa n d96021曾吉阳等:WO3气敏传感材料及器件的研究进展C o m p o u n d s,2018,768:190-197.[15]H u Y,L iL,Z h a n g L C,e ta l.D i e l e c t r i cb a r r i e rd i s-c h a r g e p l a s m a-a s s i s t ed f a b r i c a t i o n o f g-C3N4-M n3O4c o m p o s i t ef o rh i g h-p e r f o r m a n c ec a t a l u m i n e s c e n c e H2Sg a ss e n s o r[J].S e n s o r sa n d A c t u a t o r s B:C h e m i c a l,2017,239:1177-1184.[16] N a k a t eU T,A h m a dR,P a t i lP,e t a l.U l t r a t h i nN i On a n o s h e e t sf o rh i g h p e r f o r m a n c e h y d r o g e n g a ss e n s o rd e v i c e[J].A p p l i e dS u r f a c eS c i e n c e,2020,506:144971(1-8).[17] M o l a v i R,S h e i k h iM H.F a c i l ew e t c h e m i c a l s y n t h e s i s o fA l d o p e dC u On a n o l e a v e s f o r c a r b o n m o n o x i d e g a s s e n-s o r a p p l i c a t i o n s[J].M a t e r i a l sS c i e n c e i nS e m i c o n d u c t o rP r o c e s s i n g,2020,106:104767(1-9).[18] Z h a n g H,L iH R,C a iL N,e t a l.P e r f o r m a n c e so f I n-d o pe dC u O-b a s e dh e t e r o j u n c t i o n g a s s e n s o r[J].J o u r n a lo fM a t e r i a l sS c i e n c e-M a t e r i a l s i nE l e c t r o n i c s,2020,31:910-919.[19] K i mJS,Y o o nJ W,H o n g YJ,e t a l.H i g h l y s e n s i t i v ea n ds e l e c t i v e d e t e c t i o n o f p p b-l e v e l N O2u s i n g m u l t i-s h e l l e d WO3y o l k-s h e l l s p h e r e s[J].S e n s o r s a n dA c t u a-t o r sB:C h e m i c a l,2016,229:561-569. [20] K r a s n o d e b s k a-O s t r e g aB,B i e l e c k aA,B i a d u nE,e t a l.M e s o p o r o u s f i l mo fWO3-t h e"s u n l i g h t"a s s i s t e dd e c o m-p o s i t i o no f s u r f a c t a n t i nw a s t e w a t e r f o r v o l t a mm e t r i c d e-t e r m i n a t i o no fP b[J].A p p l i e dS u r f a c eS c i e n c e,2016,388:746-752.[21] W o o d w a r dP M,S l e i g h tA W,V o g t T.S t r u c t u r e r e f i n e-m e n t o f t r i c l i n i c t u n g s t e n t r i o x i d e[J].J o u r n a l o f P h y s i c sa n dC h e m i s t r y o f S o l i d s,1995,56(10):1305-1315.[22] D a sS,J a y a r a m a nV.S n O2:a c o m p r e h e n s i v e r e v i e wo ns t r u c t u r e sa n d g a ss e n s o r s[J].P r o g r e s si n M a t e r i a l sS c i e n c e,2014,66:112-255.[23]Y i n L,C h e n D,H u M,e ta l.M i c r o w a v e-a s s i s t e dg r o w t ho f I n2O3n a n o p a r t i c l e s o nWO3n a n o p l a t e s t o i m-p r o v eH2S-s e n s i n g p e r f o r m a n c e[J].J o u r n a l o fM a t e r i a l sC h e m i s t r y A,2014,2(44):18867-18874.[24] H a nX,H a nX,L iL,e t a l.C o n t r o l l i n g t h em o r p h o l o-g i e s o fWO3p a r t i c l e s a n d t u n i n g t h e g a s s e n s i n gp r o p e r-t i e s[J].N e w J o u r n a lo fC h e m i s t r y,2012,36(11):2205-2208.[25]J i aQ,J iH,W a n g D,e t a l.E x p o s e d f a c e t s i n d u c e de n-h a n c e da c e t o n es e l e c t i v es e n s i n gp r o p e r t y o fn a n o s t r u c-t u r e d t u n g s t e n o x i d e[J].J o u r n a l o fM a t e r i a l sC h e m i s t r yA,2014,2(33):13602-13611.[26] T i a nF H,G o n g C,W uR,e t a l.O x y g e nd e n s i t y d o m i-n a t e d g a ss e n s i n g m e c h a n i s m o r i g i n a t e df r o m C O a d-s o r p t i o no n t h e h e x a g o n a lWO3(001)s u r f a c e[J].M a t e-r i a l sT o d a y C h e m i s t r y,2018,9:28-33. [27] A k a m a t s uT,I t o hT,I z uN,e t a l.N Oa n dN O2s e n s i n gp r o p e r t i e so f WO3a n d C o3O4b a s e d g a ss e n s o r s[J].S e n s o r s,2013,13(9):12467-12481.[28] L i F,G u oS,S h e nJ,e t a l.X y l e n e g a s s e n s o rb a s e do nA u-l o a d e dWO3㊃H2On a n o c u b e sw i t h e n h a n c e d s e n s i n gp e r f o r m a n c e[J].S e n s o r sa n d A c t u a t o r sB:C h e m i c a l,2017,238:364-373.[29] L iY,H u aZ,W uY,e t a l.M o d i f i e d i m p r e g n a t i o ns y n-t h e s i s o fR u-l o a d e d WO3n a n o p a r t i c l e s f o r a c e t o n e s e n s-i n g[J].S e n s o r s a n dA c t u a t o r s B:C h e m i c a l,2018,265:249-256.[30] Q i nY,S h e n W,L iX,e t a l.E f f e c t o f a n n e a l i n g o nm i-c r o s t r u c t u r e a n dN O2-s e n s i n gp r o p e r t i e s o f t u n g s t e no x-i d en a n o w i r e ss y n t h e s i z e db y s o l v o t h e r m a lm e t h o d[J].S e n s o r sa n d A c t u a t o r s B:C h e m i c a l,2011,155(2):646-652.[31] H eM,X i eL,Z h a oX,e t a l.H i g h l y s e n s i t i v e a n d s e l e c-t i v e H2S g a ss e n s o r sb a s e d o nf l o w e r-l i k e WO3/C u Oc o m p o s i t e s o p e r a t i n g a tl o w/r o o m t e m p e r a t u r e[J].J o u r n a l o fA l l o y s a n dC o m p o u n d s,2019,788:36-43.[32] N a m B,K oTK,H y u nSK,e t a l.S e n s i t i v i t i e s o f a6:4(b y m o l a r r a t i o)Z n O/WO3c o m p o s i t en a n o p a r t i c l e s e n-s o r t o r e d u c i n g a n do x i d i z i n gg a s e s[J].A p p l i e dS u r f a c eS c i e n c e,2020,504:144104(1-10).[33] C h o i S,B o n y a n iM,S u nGJ,e t a l.C r2O3n a n o p a r t i c l e-f u n c t i o n a l i z e dWO3n a n o r o d s f o r e t h a n o lg a s s e n s o r s[J].A p p l i e dS u r f a c eS c i e n c e,2018,432:241-249.[34] Q i nY,Z h a n g X,L i uY,e t a l.H i g h l y a l i g n e da r r a y o fW18O49/C u Oc o r e-s h e l l n a n o r o d s a n d i t s p r o m i s i n g N O2s e n s i n gp r o p e r t i e s[J].J o u r n a lo f A l l o y s a n d C o m-p o u n d s,2016,673:364-371.[35] F e n g C,J i a n g Z,W u J,e t a l.P t-C r2O3-WO3c o m p o s i t en a n o f i b e r s a s g a s s e n s o r s f o r u l t r a-h i g hs e n s i t i v e a n d s e-l e c t i v ex y l e n ed e t e c t i o n[J].S e n s o r sa n d A c t u a t o r sB:C h e m i c a l,2019,300:127008(1-8).[36] K o o W T,C h o i S J,K i m N H,e t a l.C a t a l y s t-d e c o r a t e dh o l l o w WO3n a n o t u b e su s i n g l a y e r-b y-l a y e rs e l f-a s s e m-b l y o n p o l y m e r i cn a n o f i b e r t e m p l a t e sa n dt h e i ra p p l ic a-t i o n i ne x h a l e db r e a t h s e n s o r[J].S e n s o r s a n dA c t u a t o r sB:C h e m i c a l,2016,223:301-310.[37] D i n g X,Z e n g D,Z h a n g S,e t a l.C-d o p e d WO3m i c r o-t u b e s a s s e m b l e d b y n a n o p a r t i c l e sw i t hu l t r a h i g h s e n s i t i v-i t y t o t o l u e n e a t l o wo p e r a t i n g t e m p e r a t u r e[J].S e n s o r sa n dA c t u a t o r sB:C h e m i c a l,2011,155(1):86-92.[38] P a r kS,K i m H,J i nC,e t a l.E n h a n c e dC O g a s s e n s i n gp r o p e r t i e s o fP t-f u n c t i o n a l i z e d WO3n a n o r o d s[J].T h e r-m o c h i m i c aA c t a,2012,542:69-73.[39] S h e n d a g e SS,P a t i l VL,V a n a l a k a r SA,e t a l.S e n s i t i v ea n d s e l e c t i v eN O2g a ss e n s o rb a s e do n WO3n a n o p l a t e s[J].S e n s o r sa n d A c t u a t o r sB:C h e m i c a l,2017,240:426-433.[40] B o u d i b aA,Z h a n g C,B i t t e n c o u r tC,e t a l.S O2g a s s e n-s o r sb a s e do n WO3n a n o s t r u c t u r e s w i t hd i f f e r e n t m o r-p h o l o g i e s[J].P r o c e d i a E n g i n e e r i n g,2012,47:1033-1036.[41] T a nY,L e iY.A t o m i c l a y e r d e p o s i t i o no fR hn a n o p a r t i-c l e so n WO3t h i nf i l m f o r C H4g a ss e n s i n g w i t he n-h a n c e dd e t e c t i o nc h a r a c t e r i s t i c s[J].C e r a m i c sI n t e r n a-t i o n a l,2020,46(7):9936-9942070212020年第12期(51)卷[42] P e a r c eR ,I a k i m o vT ,A n d e r s s o n M ,e t a l .E p i t a x i a l l yg r o w n g r a ph e n e b a s e d g a ss e n s o r sf o r u l t r as e n s i t i v e N O 2d e t e c t i o n [J ].S e n s o r s a n dA c t u a t o r sB :C h e m i c a l,2011,155(2):451-455.[43] V i j a y a k u m a r S ,V a d i v e l S .F i b e r o p t i c e t h a n o l ga s s e n s o rb a s e d WO 3a n d WO 3/g C 3N 4n a n oc o m p o s i t e sb y an o v e l m i c r o w a v e t e c h n i q u e [J ].O p t i c s &L a s e rT e c h n o l o g y,2019,118:44-51.[44] W a n g C ,S u n R ,L iX ,e ta l .H i e r a r c h i c a l f l o w e r -l i k e WO 3n a n o s t r u c t u r e s a n d t h e i r g a s s e n s i n g p r o p e r t i e s [J ].S e n s o r sa n d A c t u a t o r sB :C h e m i c a l ,2014,204:224-230.[45] J i eX ,Z e n g D ,Z h a n g J ,e t a l .G r a p h e n e -w r a p pe d WO 3n a n o s p h e r e s w i t h r o o m -t e m p e r a t u r e N O 2s e n s i n g i n -d u c e db y i n t e rf a c e c h a r ge t r a n sf e r [J ].S e n s o r sa n dA c -t u a t o r sB :C h e m i c a l ,2015,220:201-209.[46] M a J ,R e nY ,Z h o uX ,e t a l .P t n a n o pa r t i c l e s s e n s i t i z e d o r d e r e d m e s o p o r o u s WO 3se m i c o n d u c t o r :g a ss e n s i n g p e rf o r m a n c e a n dm e c h a n i s ms t u d y [J ].A d v a n c e dF u n c -t i o n a lM a t e r i a l s ,2017,28(6):1705268.[47] Z e n g W ,D o n g C ,M i a oB ,e t a l .P r e p a r a t i o n ,c h a r a c t e r -i z a t i o n a n d g a ss e n s i n gp r o p e r t i e so f s u b -m i c r o n p o r o u s WO 3s p h e r e s [J ].M a t e r i a l sL e t t e r s ,2014,117:41-44.[48] L e eCY ,K i mSJ ,H w a n g I S ,e t a l .G l u c o s e -m e d i a t e d h y d r o t h e r m a l s y n t h e s i s a n d g a s s e n s i n g ch a r a c t e r i s t i c s o f WO 3ho l l o w m i c r o s p h e r e s [J ].S e n s o r sa n d A c t u a t o r s B :C h e m i c a l ,2009,142(1):236-242.[49] W a n g XX ,T i a nK ,L iH Y ,e t a l .B i o -t e m pl a t e d f a b r i -c a t i o no fh i e r a r c h i c a l l yp o r o u s WO 3m i c r o s ph e r e sf r o m l o t u s p o l l e n sf o r N O g a ss e n s i n g a tl o w t e m pe r a t u r e s [J ].R s cA d v a n c e s ,2015,5(37):29428-29432.[50] W uCH ,Z h uZ ,H u a n g SY ,e t a l .P r e p a r a t i o n of pa l l a -d i u m -d o p e dm e s o p o r o u s WO 3f o rh y d r o g e n g a ss e n s o r s [J ].J o u r n a l o fA l l o y s a n dC o m po u n d s ,2019,776:965-973.[51] Z h u Y ,W a n g H ,L i uJ ,e ta l .H i g h -pe rf o r m a n c eg a s s e n s o r sb a s e do nth e WO 3-S n O 2na n o s p h e r ec o m p o s i t e s [J ].J o u r n a l o fA l l o y s a n dC o m p o u n d s ,2019,782:789-795.[52] K o s k e l a J ,S a r f r a z J ,I h a l a i n e nP ,e t a l .M o n i t o r i n g t h e q u a l i t y o f r a w p o u l t r y b y d e t e c t i n g h y d r o ge n s u lf i d ew i t h pr i n t e ds e n s o r s [J ].S e n s o r sa n d A c t u a t o r sB :C h e m i -c a l ,2015,218:89-96.[53] L i nY ,H u a n g L ,C h e nL ,e t a l .F u l l yg r a v u r e -pr i n t e d N O 2g a s s e n s o r o n a p o l y i m i d e f o i l u s i n g WO 3-P E D O T :P S Sn a n o c o m p o s i t e s a n dA g el e c t r o d e s [J ].S e n s o r s a n d A c t u a t o r sB :C h e m i c a l ,2015,216:176-183.[54] L i S ,L i nP ,Z h a oL ,e t a l .T h e r o o mt e m pe r a t u r e g a s s e n s o r b a s e do nP o l y a n i l i n e @f l o w e r -l i k e WO 3n a n o c o m -p o s i t e sa n df l e x i b l e P E T s u b s t r a t ef o r N H 3d e t e c t i o n [J ].S e n s o r sa n d A c t u a t o r sB :C h e m i c a l ,2018,259:505-513.[55] S u n J ,S h uX ,T i a nY ,e t a l .P r e p a r a t i o no f p o l y p yr r o l e @WO 3hy b r i d sw i t h p -nh e t e r o j u n c t i o n a n d s e n s i n gp e r -f o r m a n c e t o t r i e t h y l a m i n e a t r o o mt e m p e r a t u r e [J ].S e n -s o r s a n dA c t u a t o r sB :C h e m i c a l ,2017,238:510-517.R e s e a r c h p r o g r e s s o fW O 3g a s s e n s i n g ma t e r i a l s a n dd e v i c e s Z E N GJ i y a n g ,R O N G Q i a n ,D E N G X i y u ,K U A N G X i n y a ,Z IB a o y e ,MA Y i w e n ,L I U Q i n g ju (N a t i o n a l C e n t e r f o r I n t e r n a t i o n a lR e s e a r c ho nP h o t o e l e c t r i c a n dE n e r g y M a t e r i a l sY u n n a nK e yL a b o r a t o r y o fM i c r o -n a n oM a t e r i a l s a n dT e c h n o l o g y ,S c h o o l o fM a t e r i a l s a n dE n e r g y ,Y u n n a nU n i v e r s i t y,K u n m i n g 650091,C h i n a )A b s t r a c t :W i t h t h ed e v e l o p m e n t o f h u m a nb e i n g s a n d t h e p r o g r e s s o f s o c i e t y ,p e o p l e p a y mo r e a n dm o r e a t t e n -t i o n t o t h e e n v i r o n m e n t a l p r o b l e m s ,e s p e c i a l l y t h e d e t e c t i o n o f t o x i c a n d h a r m f u l g a s e s .M e t a l o x i d e g a s s e n s o r s c a n s o l v e t h i s p r o b l e m.M e t a l o x i d e s e m i c o n d u c t o r g a s s e n s o r h a s b e e nw i d e l y s t u d i e d a n d a p pl i e db e c a u s e o f i t s s m a l l s i z e ,l o wc o s t ,c o n v e n i e n t u s e a n d q u i c k r e s p o n s e .T u n g s t e n t r i o x i d e (WO 3),a s a t y p i c a l n -t y p e s e m i c o n -d u c t o r g a s s e n s i n g m a t e r i a l ,h a s a t t r a c t e dw i d e a t t e n t i o n i n t h e d e t e c t i o n o f v a r i o u s t o x i c a n dh a r m f u l ga s e s d u e t o i t su n i q u e g a ss e n s i n gp r o p e r t i e s .T h es t r u c t u r ea n d m o r p h o l o g y o f s e n s i n g m a t e r i a l s ,t h ee x p o s e dc r y s t a l f a c e t s ,t h e i n t r o d u c t i o no f o x i d e s a n dn ob l em e t a l s p l a y ak e y r o l e i n i m p r o v i n g t h e g a s s e n s i n gpe rf o r m a n c e o f m a t e r i a l s .T h e r e f o r e ,i n t h i s p a p e r ,t h e r e c e n t s t u d i e so nt h e s yn t h e s i s ,i n t e r f a c e c o n t r o l ,m o d i f i c a t i o n m e t h -o d s ,g a s s e n s i n gp r o pe r t i e s a n d r e l a t e dm e c h a n i s m s of o n e -d i m e n s i o n a l ,t w o -d i m e n s i o n a l a n d t h r e e -d i m e n s i o n a l WO 3m a t e r i a l sw e r e s u mm a r i z e d ,t h e e x i s t i n gp r o b l e m s i n t h e r e s e a r c h p r o c e s s o fg a s s e n s o rb a s e do n WO 3at p r e s e n tw e r e p u t f o r w a r d ,a n d i t s f u t u r e d e v e l o p m e n t t r e n dw a s p r o s p e c t e d .K e y w o r d s :g a s s e n s o r ;t u n g s t e n t r i o x i d e ;n a n o s t r u c t u r e s ;g a s s e n s i n gpe rf o r m a n c e 17021曾吉阳等:WO 3气敏传感材料及器件的研究进展。
材料的气敏性能研究

材料的气敏性能研究随着科技的不断发展,气体检测和传感技术在各个领域中扮演着越来越重要的角色。
而材料的气敏性能就是其中一个关键的研究方向。
本文将探讨材料的气敏性能研究及其应用前景。
一、什么是气敏性能材料的气敏性能是指材料对气体分子的感应和响应特性。
气敏材料通常能够与气体分子发生特定的相互作用,从而导致电阻、电容、电感等物理量发生变化,进而实现对气体的检测和传感。
具有较强气敏性能的材料在环境监测、工业控制、生物医学、能源等领域有着广泛的应用。
二、气敏性能的研究方法1. 实验方法气敏性能的研究通常需要通过实验方法来获取数据。
常用的方法包括电学测量、热学测量、光学测量等。
例如,通过在气敏材料上加电,测量电阻的变化,可以获得材料对气体的响应情况。
通过控制温度,测量热敏电阻的变化,可以研究材料对不同温度下气体的敏感性。
这些实验方法可以提供基础数据,为进一步的研究和应用奠定基础。
2. 理论模拟除了实验方法,理论模拟也是研究材料气敏性能的重要手段。
通过建立合适的数学模型,可以预测材料对不同气体的响应行为,并解释实验数据的变化趋势。
理论模拟可以帮助研究者更好地理解气敏性能的原理,为材料设计和优化提供指导。
三、气敏材料的研究进展1. 传统气敏材料传统气敏材料主要包括金属氧化物、半导体等。
以金属氧化物为例,如二氧化锡、氧化锌等,这些材料具有良好的气敏性能,对多种气体有着较强的响应能力。
然而,传统气敏材料往往存在响应速度慢、抗干扰性差等不足之处,限制了其应用范围。
2. 新型气敏材料近年来,随着纳米技术的进步和材料科学的发展,新型气敏材料不断涌现。
例如,基于碳纳米管、石墨烯等二维材料的气敏材料,具有较高的比表面积和导电性,可以实现更灵敏的气体检测。
此外,功能化金属有机框架、共价有机框架等新型材料也被广泛应用于气敏传感领域。
这些新型气敏材料具有响应速度快、选择性好、抗干扰性强等优点,被认为是未来气体传感技术的重要发展方向。
三氧化钨
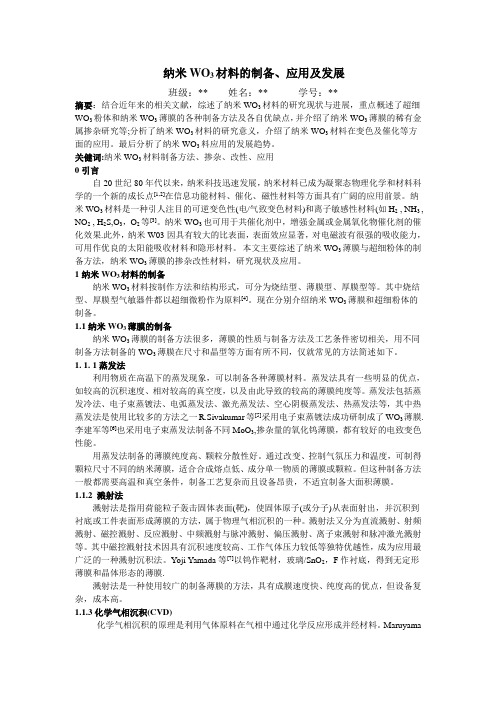
纳米WO3材料的制备、应用及发展班级:** 姓名:** 学号:**摘要:结合近年来的相关文献,综述了纳米WO3材料的研究现状与进展,重点概述了超细WO3粉体和纳米WO3薄膜的各种制备方法及各自优缺点,并介绍了纳米WO3薄膜的稀有金属掺杂研究等;分析了纳米WO3材料的研究意义,介绍了纳米WO3材料在变色及催化等方面的应用。
最后分析了纳米WO3料应用的发展趋势。
关健词:纳米WO3材料制备方法、掺杂、改性、应用0引言自20世纪80年代以来,纳米科技迅速发展,纳米材料已成为凝聚态物理化学和材料科学的一个新的成长点[1,2]在信息功能材料、催化、磁性材料等方面具有广阔的应用前景。
纳米WO3材料是一种引人注目的可逆变色性(电/气致变色材料)和离子敏感性材料(如H2 , NH3 , NO2 , H2S,O3,O2等[3]。
纳米WO3也可用于共催化剂中,增强金属或金属氧化物催化剂的催化效果.此外,纳米W03因具有较大的比表面,表面效应显著,对电磁波有很强的吸收能力,可用作优良的太阳能吸收材料和隐形材料。
本文主要综述了纳米WO3薄膜与超细粉体的制备方法,纳米WO3薄膜的掺杂改性材料,研究现状及应用。
1纳米WO3材料的制备纳米WO3材料按制作方法和结构形式,可分为烧结型、薄膜型、厚膜型等。
其中烧结型、厚膜型气敏器件都以超细微粉作为原料[4]。
现在分别介绍纳米WO3薄膜和超细粉体的制备。
1.1纳米WO3薄膜的制备纳米WO3薄膜的制备方法很多,薄膜的性质与制备方法及工艺条件密切相关,用不同制备方法制备的WO3薄膜在尺寸和晶型等方面有所不同,仅就常见的方法简述如下。
1. 1. 1蒸发法利用物质在高温下的蒸发现象,可以制备各种薄膜材料。
蒸发法具有一些明显的优点,如较高的沉积速度、相对较高的真空度,以及由此导致的较高的薄膜纯度等。
蒸发法包括蒸发冷法、电子束蒸镀法、电弧蒸发法、激光蒸发法、空心阴极蒸发法、热蒸发法等,其中热蒸发法是使用比较多的方法之一R.Sivakumar等[5]采用电子束蒸镀法成功研制成了WO3薄膜.李建军等[6]也采用电子束蒸发法制备不同MoO3,掺杂量的氧化钨薄膜,都有较好的电致变色性能。
WO_3基气敏传感器

工作时是检测 NOx 的高敏感材 料。随后的两年里 人们研究了诸如半导体型、电化学型等各种类型的 NO2 气敏传感器[ 24 ) 28] , 并一致认为 WO3 是最具有前 景的 NO2 气敏传感器[ 29 ) 33] 。
1995 年, Inoue 等[34] 通 过 在 空 气 中 加 热 分 解 ( NH4 ) 10 W12O41 制备了 WO3 , 并采用丝网印刷法制备 了 WO3 厚膜型 NO2 气敏传感器, 该传感器可以检测
# 2736 #
化学进展
第 21 卷
出 2 ) 3ppm NO2 气 体, 而 且 稳 定 性 良 好。同 年, Sberveglieri[ 35] 等采用磁控溅射法制备了 WO3 薄膜, 并研 究 了 其 在 200 ) 500 e 的 温 度 范 围 内 对 1 ) 10ppm NOx 的敏感特性。结果表明, 该薄膜 在上述 条件下对 1 ) 10ppm 的 NOx 具有良好的重复性和气 敏响应, 并可以选择 性地检测出 CH4、CO 和 SO2 气 体, 其在 400 e 时对 10ppm NOx 的灵敏度为 118, 但 是该 研究 并 没有 探讨 其 气敏 机 理。1996 年, Nelli 等[ 36] 研究了纳米尺寸的 T-i W 氧化物薄膜对 015 ) 20ppm 的乙醇气体、CH4、CO 和 NO2 的气敏性能。结 果表明, 在上述气体中敏感膜对 NO2 的敏感特性最 好, 在最佳工作温度 300 e 时敏感膜对 20ppm NO2 的 灵敏度为 20。1997 年, Penza 等[ 37] 利用 WO3 薄膜作 敏感 涂层制备了表面声波 NOx 气敏传感器。1998 年, 该研究组利用 磁控溅射法制 备了 WO3 薄膜 型 NOx 气 敏传 感器, 同 时, 还 研究 了该 薄膜 对 NH3 、 H2 S、SO2 、CO 和 CH4 的敏感性 能。研究 结果表明, WO3 薄膜在 250 e 时对 NO x 具有良好的敏感特性, 其响应时间在 60s 以内[ 38] 。该研究组还研究了 Pd、 Pt、Au 掺杂的 WO3 薄膜对 NOx 的敏感性能[ 39] 。同 年, Kim[40] 等研 究了 WO3 薄膜 沉积 方式 的 变化 对 NOx 气敏特性的影响。Tomchenko 等[ 41] 则采用丝网 印刷法制备了 Bi2 O3 掺杂的 WO3 厚膜气敏传感器。 结果表明, 掺杂 Bi2O3 且厚度为 25Lm 的WO3 敏感膜 在 300 e 时对 2 ) 300ppm 的 NO 具有良好的气敏性 能, 在 该 温 度 下 对 163ppm NO 气 体 的 灵 敏 度 为 2196, 响应、恢复时 间分别为 214min 和 7min。1999 年, Lee 等[42] 、Chung 等[ 43] 、Yang 等[ 44] 和 Tomchenko 等[ 45] 先后研究了 WO3 基纳米晶和厚膜气敏传感器 对 NOx 的敏感特性。例如, Chung 等[ 43] 通过改变制 备工艺条件( 烧结温度、退火气 氛等) , 研究了 WO3 厚膜型 NO2 气敏传感器的敏感特性。结果表明, 经 700 e 烧结的 WO3 厚膜在低温 ( 100 e ) 时 对 NO2 气 体具有良好的气敏性能。Yang 等[44] 则详细研究了 黏结剂聚乙二醇( PVA ) 、Si 溶胶和 A l2O3 添加 剂对 NOx 敏感性能的影响。研究结果指出, 添加剂等对 WO3 基气敏传感器气敏性 能的影响主要是通 过对 晶界的修饰实现的, 而不是通过改变晶粒尺寸或者 电子浓度实现的。
- 1、下载文档前请自行甄别文档内容的完整性,平台不提供额外的编辑、内容补充、找答案等附加服务。
- 2、"仅部分预览"的文档,不可在线预览部分如存在完整性等问题,可反馈申请退款(可完整预览的文档不适用该条件!)。
- 3、如文档侵犯您的权益,请联系客服反馈,我们会尽快为您处理(人工客服工作时间:9:00-18:30)。
[] 尼科利斯,. 3 G. I普里戈京著 , 徐锡 申等译 . 非平衡系统 的自组织, 北京 : 科学 出版社 , 8 :9 — 0 . 1 646 50 9
中 图分 类号 :P222 T 1. 文 献标 识码 : A
用于检测微量 Hs 2气体的 S0 元件具有相当 n2 高的灵敏度 , 但响应- 恢复时间较长, 选择性的提高 也受限制 。本 文用 化 学沉淀法 合成 了 x t%S 2 w. i 一 0 wo( = ,,,01)  ̄x 035 1,5纳米粉体, 研制出一系列烧
图 3 元 件 的 气敏 性 能与 工作 温度 的 关 系
Fg 3 S n ivt f8,sr .a i . e st i o no 8 i y e s 8 fn t no okn e e aue u c o fw rig tmp rtr i
从 图 3 可 以看 出 , 温度条 件相 同 的情 况 还 在 下, 掺杂有 So 的气敏元件 均 比纯 wo 气敏 元件 i2 3 对 H S气 体 的灵 敏 度 有 所 提 高 , 中掺 杂 量 为 2 其 5 %时 , 增敏现象 最为 突出 ,8 o该元 件对体积分 10C 参考文献 :
( 下转第 30页 ) 8
维普资讯
30 8
化 学研 究 与应 用
第l 6卷
在培养基 中央 , 现细菌 在接种 8h后 开始波 动 , 发 在接 种 2 后观测结 果列于表 中。 6h 表 细菌被接 种 2 后的实验观测 结果 6h
T be E p r na a l x e me tl ̄s t fb ceim i l o u a tr u pa td atr2 ln e f 6 h. e
一
化趋势 。从而为我们 以系统动力学研 究方 法来揭 示生物体 系中群体数量 的演化 问题提供有益参考 。
通过以上群体演变动力学方程的建立和数值 模拟以及对该体系所进行的实验观察研究 , 发现 该群体演变的模型及反应扩散方程 , 经过数值模 拟分析后能够较好解释我们在奇异变形杆菌波动 过程 中所观察到 的奇异变形杆 菌活菌数的动态变
维普资讯
化 学 研 究 与 应 用
C e c l sac n pia o h mia e rh ad Ap l t n Re ci
Vo. 6. o. 11 N 3 Jn. 2 u .a
文章编 号 :0 415 {0 4 0—370 10 — 6 20 )307—3 6
=2 — 5范 围内最强峰 的相对 强度 可 以看 出 , 2 2。 当
图 2中 ab分别 为纯 wo 粉体及掺杂 比例 、 3 为 5 i2 3 %S 一 粉体 的 T M照片 , 0 wo E 放大倍数为 5 万倍, 比例计算后可知 , wo 粉体 的平均粒 按 纯 3 径大约为 4 n,2 i2 3 4nl5 %S 一 o wo 粉体的平均粒径 为 3 . nl 25 n, 粒度均匀 。 22 材料 的气敏 性能 . 由图 3 可知, 元件 的灵敏度先是随着工作 温 度的升高逐渐增大 , 当工作温度为 10I时, 8c = 各元 件的灵敏度达到该掺杂条件下的最大值 , 随后当
=
上 30I 老化 20h 2 c下 = 4 。
气敏性能的测试采用静态配气法, 测试气体 有 H S N-、 H O 、 酮 、 2、 I 5 H 丙 1 3 汽油 、 甲苯等 , 元件 的 加热电压可在较大范围内调节 , 负载电阻为可换 插 卡式 , 义 元件 的灵 敏 度 S=R / 。 R 、 g 定 a船 a R 分别为元件在空气和被测气体中的电阻。
38 7
化 学研 究与应 用
第 1 卷 6
a
图 2 S 2Wo 粉体 的 T M 照片 i- 3 o E
Fg. T M ma e fSO2W O3p w es i 2 E i g so i - o d r
温度 继续升 高 时 , 元件 灵敏 度 开始 降低 。这 是 由 于元件 的气 敏性 能 不仅 与基 体 材料 有关 , 同气 还 体 与材料 的表 面作 用息息相关 【 。元件工作温度 2 J 较低 时元件 的表 面活性较低 , 化学 吸附氧太少 , 与 气体 的作用较弱 , 因而 灵敏度较低 ; 由于 H s 2 是一 种强还原性 气 体 , 温度 过 高时 元件 表面 的氧 化反 应速 度过快会 限制 H S气体 的扩 散 , 得元件 表 2 使 面被测 气体 的浓 度很小 , 会引起灵敏度降低 。 同样 该元件 较其它 H S气敏元件 的最佳 工作 温度有很 2 大降 低 , 元件 工作 温度 越低 , 与环境 温差越 小 , 元 件 的阻值受 环境温 度的影响越小 , 实用性越强Байду номын сангаас 。 3
掺杂量 为 3 5 1 %时 , 强 峰 在 左 侧 , 、、0 最 当掺 杂 量 为 1%时最强峰移至右侧 , 5 这是因为掺杂量不同 时 , 在 wo 晶格 内 的分 布有所 不 同 , S 3 当掺 杂
量增加到一定程度时 , 3 wo 的结合状态发生 了变 化, 出现一定的定 向性。随着掺杂量的增加, R XD 结型气敏元件, 工作温度为 1  ̄ 掺杂量为 5 8 C, 0 %的 其中 1%s 2 3图谱的 5 i一 o wo 气敏元件对 Hs 2 有很高的灵敏度和选择性, 响应一 谱有一定的宽化现象 , 宽化现象较为明显 , 这是 由于 S 的掺杂在一定 恢复快 , 而且工作温度较低 , 有较好的应用价值。 程度上抑制 了 wo 晶粒 的生长 , 3 随着 掺杂 量 的增 1 实验方法 加, 粉体粒径变小而引起的。由 Se e公式计算 hrr r 得粉体的晶粒尺寸 2 .4 4 .8nl X D谱一 13 — 22 n与 R 1 1 气敏材料 的合成 . 在一定 量 的钨 酸铵 ( 学纯 ) 化 中加去 离子 水 , 致 。 加热至 6 c使其溶解 , oI = 在此条件 下将 正硅酸乙 酯、 无水乙醇、 盐酸 ( 分析纯) 比例混合加入其 按 中, 不断搅拌 , 形成 黄色沉淀 , 过滤、 洗涤、 烘干、 60C 0  ̄高温下煅烧 5h 自然冷却, , 研磨制得 xt% w. (,,, ,5 的 s 2 3 0351 1) i . 气敏粉体材料。 0 0 wo 12 气敏元 件的制作及气敏性 能测试 . 取 s 2 3 体材料 少许 , i一 粉 0 WO 加入粘合 剂磨 匀 , 到已制好 的带有 金 电极 和 铂 引线 的氧化 铝 涂 细瓷管上 , 烘干、0c下焙烧 1h冷却后在瓷管 6oI = , 中间串入电阻丝并焊接到六腿管座上, 在老化台
数为 00 5 E 气 体灵敏度达到 了 9 , . %H S 0 3 是同样温 度下其 它元件 的 3~ 7倍 。这是 由于添 加适 量 的 s 抑制 了晶粒 的生长 , 减小粉 体粒径 , 增大 了比 表面 积 之 故 。但 随 着 掺 杂 量 的增 加 , 有 部 分 会 s 分布在 wo 晶粒表 面 , 碍 了晶粒表 面对 被 3 阻 测气体 的吸附一 吸过程 , 解 因而影 响材 料 的气 敏性 能。尽 管掺 杂量为 1% 、5 0 1%时粉 体粒 径 比掺 杂 量为 5 %时小 , 但其 气 敏性 能 却 降低 了 , 明 5 说 % 的掺杂 为最佳掺 杂量 。 气敏元件在 相同环境 中对被 检测 气体 有较好 的灵敏度 , 其它 气体 没有 灵 敏度 或灵 敏 度较 小 对 选择性较好 。最佳 掺杂量 5 i2 3 敏元 件 %S0一 wo 气 10 8 ℃下对 H S 丙酮 、 2、 汽油 、 甲苯 、 精 、 气等 六 酒 氨 种气体 的灵敏 度 , 中 , 酮 、 其 丙 氨气 的体积 分数 为 00% , .1 其余气体体积分数 为 0 05 .0 %。该 元件 对 H s 灵敏度 为 9 ) 2( 3 以外 的几 种 气 体 灵 敏度 很 小 , 其 抗干扰能力强 , 选择性很好 。 响应时 间 £ 为元件接触被 测气体后 , 载 电 负 阻 R. 的 电压 由 I 上 变 化 到 +9 % ( x U ) O U— 0 所 需的时间 , 复 时 间 tv 元 件脱 离被 测 气 体 恢 为 r e 后 , 载 电阻 R 的 电压 由 负 L 恢 复 到 +1 % 0 ( xU ) U — 0所用 的时间 , 为元件 在 空气 中时负 载 电阻上的电压值 , 为元 件在被测气 体 中时负载 电阻上 的电压值 。环境 温 度 3 c , 度 5 %R O【 湿 = 0 H, 为 10【5 S 2 3气 敏 元 件 对 体 积 分 数 为 8c % i 一 = 0 wo 00 5 2 气体 的响应一 复 曲线 显 示 元 件接 触 . %H S 0 恢 被测气体后 , 阻值降低 , 负载 电压升高 , 响应较快 , 反之 , 离被测气体后 , 脱 负载 电压降低 , 电阻升 高 。 计算得 响应 时间 is 5s恢 复时 间 t =2 , = , r e 3s说 明元件 响应一 恢复较快 , 有较好 的实用价值 。
WO 纳 米 材 料 的 H S气 敏 特 性 3 2
魏少红 张 岩2牛新书 , ,
(. 1安阳师范学院化学系, 河南 安阳 450 ;. 5002平顶山工学院化学系, 河南 平顶 山 470 ; 600 3河南 师范大学化学与环境科学学 院 , . 河南 新乡 4 30 ) 502
关键词 : 三氧化钨 ; 硫化氢; 气敏特性
[] 1 钱逸泰, 结晶化学导论 , 合肥 : 中国科技大学 出版社 , 19 : 6 7 . 992 —27 7
37 7 . 6 —30 [] 3 全宝富, 孙良彦, 吴家琨 , 传感器技术, 9, )1—1. 1 6( : 9 3 5 9
[] 2 徐甲强, 刘艳丽, 牛新书, 无机材料学报, 0 , () 2 21 2 : 0 7
参 考文献 :
[] 玖平 , 久里 , 1徐 罗 徐启 旺 . I 四川 大学学 报 ( 自然科 学