地铁地表沉降外文翻译(适用于毕业论文外文翻译+中英文对照)
地铁隧道施工外文文献翻译
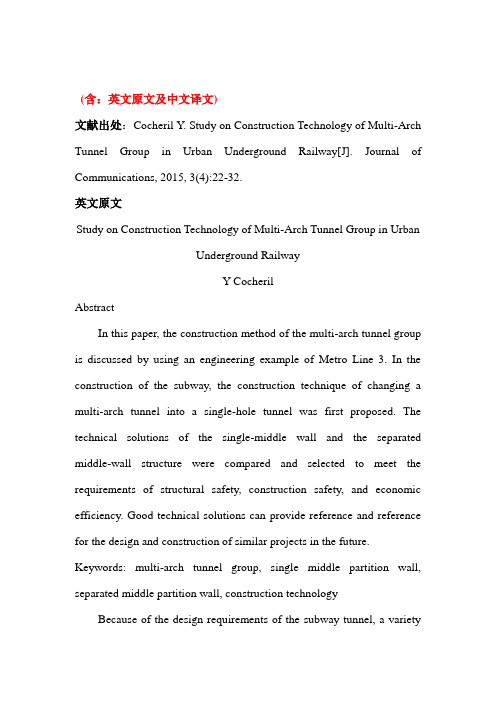
(含:英文原文及中文译文)文献出处:Cocheril Y. Study on Construction Technology of Multi-Arch Tunnel Group in Urban Underground Railway[J]. Journal of Communications, 2015, 3(4):22-32.英文原文Study on Construction Technology of Multi-Arch Tunnel Group in UrbanUnderground RailwayY CocherilAbstractIn this paper, the construction method of the multi-arch tunnel group is discussed by using an engineering example of Metro Line 3. In the construction of the subway, the construction technique of changing a multi-arch tunnel into a single-hole tunnel was first proposed. The technical solutions of the single-middle wall and the separated middle-wall structure were compared and selected to meet the requirements of structural safety, construction safety, and economic efficiency. Good technical solutions can provide reference and reference for the design and construction of similar projects in the future. Keywords: multi-arch tunnel group, single middle partition wall, separated middle partition wall, construction technologyBecause of the design requirements of the subway tunnel, a varietyof tunnel structures are required. Among them, a multi-arch tunnel segment consisting of unequal cross-linked arches and triple-arched tunnels is often used for the connection of the main line and the crossover line. This article combines the project example according to the geological conditions of the tunnel, the time limit requirements through comparison and selection of the best construction program that can achieve rapid construction and save construction costs.1 Project OverviewThe return line of Sports West Road Station on Metro Line 3 is a complex type of return line from Sports West Road Station. In the section DK3016.047037.157, a tunnel group with unequal spans with double arches and triple arches was set up. Unequal cross-arch tunnel excavation span of 20.1m excavation height of 10.076m cross-vector ratio of 1:0.5 hole lining after the lining of 5.2m large-hole lining after the span of 11.4m in the wall thickness of 1.6m. The triple-arch tunnel excavation span is 19.9m and the 7.885m cross-vector ratio is 1:0.1. The surrounding rock of the section of the multi-arch tunnel is from top to bottom: artificial backfill, red sand and alluvial sand layer, alluvial-diluvial earth, fluvial-lacustrine sedimentary soil, plastic residual soil, hard plastic-hard residue. Soil, weathered rock formations, strong weathered rock formations, weathered layers, and weathered layers. Tunnels through the formation of more homogeneous rock strength, strong bearing capacityand stability. The thickness of the vault covering the tunnel is 15.518m, and the thickness of the surrounding rock layer IV is 5.67.6m. The buried depth of groundwater in the section of the multi-arch tunnel is 2.284.1m, mainly Quaternary pore water and fissure water.2 double arch construction planDue to the complex structure of the multi-arch tunnel section, the tunnel section changes greatly. The construction process is complex and the construction is difficult. The construction period is long. Therefore, it is very important to choose a good construction scheme to complete the construction of the multi-arch tunnel section with high quality and efficiency. When selecting a construction plan, the following aspects are mainly considered: 1 Construction safety and construction Safety 2 Construction difficulty 3 Construction cycle 4 Economic benefits. Based on these four principles, the following two construction plans were selected for comparative selection through the research and demonstration of the construction plan.2.1 Single Wall Construction PlanThe main construction steps and measures of this program are as follows: 1 Prevent the construction of the middle wall from timely construction after the completion of the construction of the temporary construction channel, double-arched and triple-arched intermediate wall from the double-arched tunnel on the right line to the return line side. . 2After the construction of the middle wall lining is completed, the CRD construction method for the right line shall be used for the construction of the large-span tunnel of the re-entry line in accordance with the principle of “small first, large, and closed”. (3) When the construction of the triple-arch tunnel on the side of the re-entry line is carried out, the construction of the triple-arch and double-arched middle wall shall be carried out in accordance with the construction method of the middle-wall of the right line. After the completion of the construction of the four-fold line on the side of the middle wall, the construction of the right line will continue. This construction method is applied to the general construction methods of domestic double-arch tunnels in Guangzhou Metro, Nanjing Metro and Beijing Subway, and can safely and smoothly complete the construction of tunnel groups. However, the study of previous engineering examples and construction techniques can reveal that the program still has shortcomings and defects. 1 This scheme is applied frequently in this project. The initial support and the secondary lining of the tunnel within the short 21.11m multi-arch tunnel will convert 4 times.2 The waterproof layer construction, reinforcement engineering, formwork engineering, and concrete pouring involved in the lining of the middle wall and side tunnels all require multiple conversions and a construction period of up to 2 months. After the completion of the lining, the investment of the anti-bias support of the middle wall and theequipment and equipment will lead to higher construction costs and lower economic benefits.2.2 Separated Wall Construction PlanThe main construction steps and measures of this plan are as follows: 1 Change the unequal span double-arch tunnels into two single holes to change the separation-type mid-rise wall first from the right-line single-line tunnel construction. 2 Double-arched tunnels will not be used for middle-liner lining under single-line conditions. 3 The right-sided large-section double-arch tunnel passes through the side wall of the CRD method. For the 4 fold back line, the construction is performed in the reverse order of the right line. Adopting this scheme is actually a comparison between the two single-line construction methods and the previous one. This has the following advantages: 1 Reduce the number of construction processes and speed up the transition of the process. 2 Reduced the difficulty of construction and shortened the construction period. 3 Reduced construction costs and increased economic efficiency.4 The change to a single wall in the middle of the wall has completely solved the waterproofing defects of the double-arch tunnel structure.5 The construction of the middle tunnel of a triple-arch tunnel is equivalent to a large-span tunnel with reserved core rock, which is conducive to the construction of safety double-arch tunnels on both sides.3 Three Arches Construction PlanFrom the right line directly into the triple-arch tunnel, its supporting parameters are based on the original design, and the entire ring is installed. The whole ring is sprayed on the design and the anchor bar at the middle wall is reinforced. The re-entry side is the same as the right-line construction method. It is necessary to remove a longitudinal reinforcement beam at the junction of the tunnel grille. Strictly control the distance between each step of the excavation footage grid is 0.6m/榀. The middle-wall excavation adopts a weak-weak-weakening blasting scheme to conditionally use the static blasting scheme to minimize the disturbance to the middle-wall rock formation and the lining tunnel to ensure construction safety. The secondary lining is performed immediately after the middle wall excavation is completed. After the completion of the construction of the middle wall, the gaps in the middle walls will be backfilled with jack support. Only one side of the construction is completed before the other side of the wall construction. After the completion of the construction of the middle walls on both sides, the secondary lining of the single-hole tunnels on both sides shall be promptly conducted, and then the excavation and lining of the middle rock mass of the triple-arch tunnel shall be carried out. Special attention should be paid to the settlement and convergence deformation of the triple-arch tunnel at the middle of construction.4 Analysis of structural behavior during constructionChanged the cancellation of mid-walls that do not cross double arches into separated walls. There is no similar engineering design and construction experience in domestic urban subway projects, and there is no similar tunnel structure design. Therefore, whether the structure is safe and whether the construction process is changed during the construction process. Safety will be the focus of this program. Using ANSYS finite element general program software to perform numerical simulations on unequal cross-arch tunnels. The strata-structure model was used to analyze the stress and deformation of the tunnel structure (Fig. 1, Fig. 2, Fig. 3). The horizontal direction of the force taken along the direction of the tunnel is limited to 3 times the hole span. The vertical direction is taken upwards to the surface, and the bottom is 3 times the hole span. Element model Elasto-plastic physical tunnel lining with DP stratum material adopts elasticity The beam element simulation beam elements and solid elements are connected using a coupling equation. It can be seen from the data analysis in Table 2 that the large tunnel has a greater impact on the small tunnel during construction. If the necessary reinforcement measures are taken for the small section tunnel and the longitudinal demolition distance of the temporary support is controlled, this scheme is beneficial and feasible.5 Key Construction Technologies and Corresponding MeasuresThe construction of the multi-arch tunnel section needs to be carriedout under strict construction organization and strong technical guarantee measures. The construction of each construction step is a key to successful construction.5.1 Pulling bolts and reinforcing boltsAfter the removal of the single middle wall, the thickness of the middle wall after the excavation is completed is 0.8m. It is very necessary to set the anchor bolt and the reinforcement bolt. For the tension bolt, the length of the Φ22 steel reel bolt i s 0.6m2150.5m, and the thickness of the middle wall is 0.82.0m. Reinforced anchor rods are installed at the inverting arch and side wall at both sides of the middle wall with a Φ25 hollow grouting anchor spacing of 0.6m21.50.8m.5.2 Grouting Reinforcement in Middle Wall Rock PillarThe thinnest part of the rock mass in the middle wall is 0.15m. After several blasting excavation processes, the surrounding rock around the middle wall loosens its bearing capacity. Therefore, the loose surrounding rock must be grouted in the vaults, walls and inverted arches of the middle wall. The embedded Φ42 steel pipe slurry adopts a cement-water glass double slurry parameter of 1:1 cement slurry and 3045Be. In the two excavations, the grouting pressure of the inflow glass solution of the middle wall is 0.21.0 MPa. After the final excavation of the grouting line,a saturated grouting is performed on the sandwich wall.5.3 Differential Blasting TechnologyAll the tunnel excavations are drilled and blasted. Because the ground buildings in the downtown area of Guangzhou City are dense and the tunnel is blasted at a distance of “0”, the blasting vibration must be controlled within the allowable range in accordance with the blasting scheme for micro-shock blasting in the reserved smooth layer. The blasting measures taken for Grade III and Grade IV surrounding rocks in the strata of a multi-arch tunnel are as follows: (1) Blasting equipment uses emulsion explosives with low seismic velocity. 2 Strictly control the distance between the perforation of 0.60.8m per cycle and the distance between the peripheral blastholes of 0.4m to reduce the charge volume and control the smooth blasting effect. 3 Multi-stage detonator detonation in each blasting The non-electrical millimeter detonator is used to asymmetrically detonate the network micro-vibration technology. 4Second excavation is adopted at the middle wall. 1m is reserved for the smooth surface. Grooves are arranged on the side far away from the middle wall. medicine. The use of artificial wind excavation for excavation of partially dug excavation is prohibited. Through the above-mentioned effective measures, the “0” distance excavation of the multi-arch tunnel was smoothly passed without causing damage to the 0.15-m thick middle wall during the secondary blasting of the middle wall.5.4 Assisted Scissor SupportThrough ANSYS simulation analysis In order to ensure the safety of small-section tunnel construction, it is necessary to assist the reinforcement of the small-section tunnel to withstand the transient impact caused by blasting and the bias generated by the load release during excavation of the rock formation. The support material is welded to both ends of the grid pre-embedded steel plate with I20 steel and the spacing of the support arrangement is 0.6m, ie high strength bolts are used on each grid. The layout of the arrangement was extended to 1.2m on each side of the double arch and completed in front of the big end of the excavation. The height and angle of the support arrangement should ensure smooth construction machinery and equipment. Through the construction proof that the setting of the support is necessary and effective, the small section tunnel converges only 5 mm after the auxiliary scissor is added.5.5 Information ConstructionIn order to ensure structural safety and construction safety, real-time monitoring measurement is carried out during the tunnel construction process. The deformation characteristics of supporting structures and surrounding strata are used to predict the corresponding support structure displacements and to verify the rationality of supporting structures to provide a basis for information construction. Monitoring during construction shows that the maximum settlement of a tunnel with a smallcross section is 14.6 mm. The maximum settlement of a tunnel with a large section is 17.2 mm. The maximum convergence of the tunnel is 7.6 mm. The maximum settlement of the ground is 10 mm. The maximum settlement of the arch with a triple hole arch is 22.8 mm.中文译文城市地下铁道连拱隧道群施工技术研究作者Y Cocheril摘要本文利用地铁三号线某一工程实例对连拱隧道群施工工法进行探讨。
西安工业大学建工院土木工程毕业设计总说明书外文文献翻译
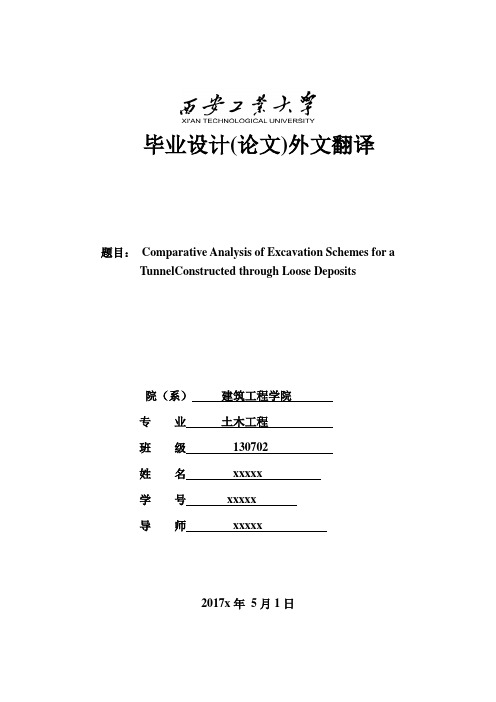
毕业设计(论文)外文翻译题目:Comparative Analysis of Excavation Schemes for a TunnelConstructed through Loose Deposits院(系)建筑工程学院专业土木工程班级130702姓名xxxxx学号xxxxx导师xxxxx2017x年5月1日通过松散堆积物构建了隧道开挖方案的对比分析摘要:由于周围岩石较弱,构造松散沉积物的隧道易于坍塌,二次内衬通常遭受过度变形。
因此,选择适当的挖掘方案是重要的,这将对隧道施工安全和随后的隧道运行产生影响。
本文采用亭子坝隧道,一条浅埋在浅沉积和冲积起源的高速公路隧道为例。
在施工期间,这条隧道经历了很多穹顶倒塌事件和先进的支援破坏。
对重组样品进行各向同性排水(CD)压缩试验,以获得松散沉积物的机械参数。
进行三维建模以模拟三种不同方案开挖后隧道中的应力和变形分布,即上下台阶隧道,三台隧道和单侧方向隧道掘进。
比较分析结果表明,单侧巷道隧道更适合该隧道,既可以减少拱顶沉降,又可以限制塑性区的开发。
对于类似地质环境中的隧道设计和施工,结果应该是重要的。
关键词:松散堆积物;力学参数; 隧道;开挖方案;比较分析。
说明随着中国交通基础设施快速发展,在过去的几十年里,许多新的隧道已经或正在通过具有挑战性的地质条件的地区建设等。
软岩在隧道建设中经常遇到。
软岩的力学特性导致快速变形和各种干扰(Sharifzadeh等人。
2013a;朱某等人。
2013)它能影响地下结构的稳定性。
为此,软岩石已受到很多关于交通隧道建设的关注。
例如,Jeng等人(2002)评价Mushan的变形砂岩和台湾北部对隧道变、形的影响。
Ozsan和Basarr(2003)计算出强风化凝灰岩Urus坝址引水隧洞的支持能力。
李和舒伯特(2008)研究了在软弱围岩中圆形隧道的长度。
Shahrour 等(2010)分析了用软土构建的隧道的地震响应。
地铁盾构隧道施工地表沉降的研究
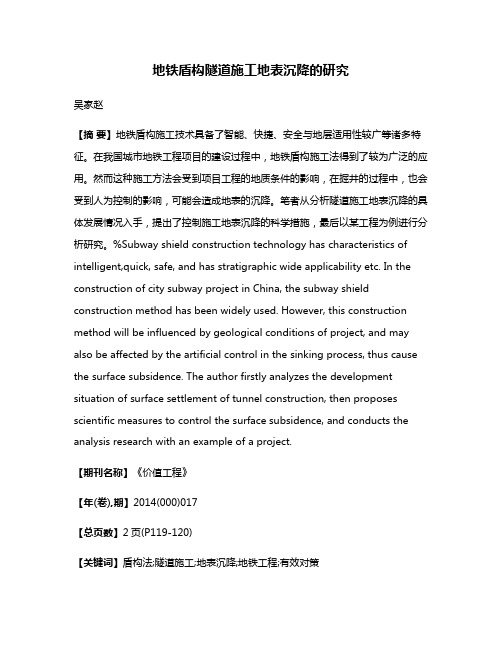
地铁盾构隧道施工地表沉降的研究吴家赵【摘要】地铁盾构施工技术具备了智能、快捷、安全与地层适用性较广等诸多特征。
在我国城市地铁工程项目的建设过程中,地铁盾构施工法得到了较为广泛的应用。
然而这种施工方法会受到项目工程的地质条件的影响,在掘井的过程中,也会受到人为控制的影响,可能会造成地表的沉降。
笔者从分析隧道施工地表沉降的具体发展情况入手,提出了控制施工地表沉降的科学措施,最后以某工程为例进行分析研究。
%Subway shield construction technology has characteristics of intelligent,quick, safe, and has stratigraphic wide applicability etc. In the construction of city subway project in China, the subway shield construction method has been widely used. However, this construction method will be influenced by geological conditions of project, and may also be affected by the artificial control in the sinking process, thus cause the surface subsidence. The author firstly analyzes the development situation of surface settlement of tunnel construction, then proposes scientific measures to control the surface subsidence, and conducts the analysis research with an example of a project.【期刊名称】《价值工程》【年(卷),期】2014(000)017【总页数】2页(P119-120)【关键词】盾构法;隧道施工;地表沉降;地铁工程;有效对策【作者】吴家赵【作者单位】中交隧道工程局有限公司,西城100088【正文语种】中文【中图分类】U4550 引言隧道技术的进一步发展,使得盾构隧道逐渐成为了繁忙闹市区或是软弱岩土层地下工程项目施工的重要施工措施。
土木工程 建筑外文翻译 --基于盾构法的Istanbul地铁施工引起的地面沉降预测

中文5351字出处:Environmental Earth Sciences, 2011, 62(2): 357-365外文原文Surface settlement predictions for Istanbul Metrotunnels excavated by EPB-TBMS. G. Ercelebi • H. Copur • I. OcakAbstract In this study, short-term surface settlements are predicted for twin tunnels, which are to be excavated in the chainage of 0 ? 850 to 0 ? 900 m between the Esenler and Kirazlı stations of the Istanbul Metro line, which is 4 km in length. The total length of the excavation line is 21.2 km between Esenler and Basaksehir. Tunnels are excavated by employing two earth pressure balance (EPB) tunnel boring machines (TBMs) that have twin tubes of 6.5 m diameter and with 14 m distance from center to center. The TBM in the right tube follows about 100 m behind the other tube. Segmental lining of 1.4 m length is currently employed as the final support. Settlement predictions are performed with finite element method by using Plaxis finite element program. Excavation, ground support and face support steps in FEM analyses are simulated as applied in the field. Predictions are performed for a typicalgeological zone, which is considered as critical in terms of surface settlement. Geology in the study area is composed of fill, very stiff clay, dense sand, very dense sand and hard clay, respectively, starting from the surface. In addition to finite element modeling, the surface settlements are also predicted by using semi-theoretical (semi-empirical) and analytical methods. The results indicate that the FE model predicts well the short-term surface settlements for a given volume loss value. The results of semi-theoretical and analytical methods are found to be in good agreement with the FE model. The results of predictions are compared and verified by field measurements. It is suggested that grouting of the excavation void should be performed as fast as possible after excavation of a section as a precaution against surface settlements during excavation. Face pressure of the TBMs should be closely monitored and adjusted for different zones.Keywords Surface settlement prediction _ Finite element method _ Analytical method _ Semi-theoretical method _ EPB-TBM tunneling _Istanbul MetroIntroductionIncreasing demand on infrastructures increases attention to shallow soft ground tunneling methods in urbanized areas. Many surface and sub-surface structures make underground construction works very delicate due to the influence of grounddeformation, which should be definitely limited/controlled to acceptable levels. Independent of theexcavation method, the short- and long-term surface and sub-surface ground deformations should be predicted and remedial precautions against any damage to existing structures planned prior to construction. Tunneling cost substantially increases due to damages to structures resulting from surface settlements, which are above tolerable limits (Bilgin et al. 2009).Basic parameters affecting the ground deformations are ground conditions, technical/environmental parameters and tunneling or construction methods (O’Reilly an d New 1982; Arioglu 1992; Karakus and Fowell 2003; Tan and Ranjit 2003; Minguez et al. 2005; Ellis 2005; Suwansawat and Einstein 2006). A thorough study of the ground by site investigations should be performed to find out the physical and mechanical properties of the ground and existence ofunderground water, as well as deformation characteristics, especially the stiffness. Technical parameters include tunnel depth and geometry, tunnel diameter–line–grade, single or double track lines and neighboring structures. The construction method, which should lead to a safe and economic project, is selected based on site characteristics and technical project constraints and should be planned so that the ground movements are limited to an acceptablelevel. Excavation method, face support pressure, advance (excavation) rate, stiffness of support system, excavation sequence and ground treatment/improvement have dramatic effects on the ground deformations occurring due to tunneling operations. The primary reason for ground movements above the tunnel, also known as surface settlements, is convergence of the ground into the tunnel after excavation, which changes the in situ stress state of the ground and results in stress relief. Convergence of the ground is also known as ground loss or volume loss. The volume of the settlement on the surface is usually assumed to be equal to the ground (volume) loss inside the tunnel (O’Reilly and New 1982).Ground loss can be classified as radial loss around the tunnel periphery and axial (face) loss at the excavation face (Attewell et al. 1986; Schmidt 1974). The exact ratio of radial and axial volume losses is not fully demonstrated or generalized in any study. However, it is possible to diminish or minimize the face loss in full-face mechanized excavations by applying a face pressure as a slurry of bentonite–water mixture or foam-processed muck. The ground loss is usually more in granular soils than in cohesive soils for similar construction conditions. The width of the settlement trough on both sides of the tunnel axis is wider in the case of cohesive soils, which means lower maximum settlement for the same amount of ground loss.Time dependency of ground behavior and existence of underground water distinguish short- and long-term settlements (Attewell et al. 1986). Short-term settlements occur during or after a few days (mostly a few weeks) of excavation, assuming that undrained soil conditions are dominant. Long-term settlements are mostly due to creep, stress redistribution and consolidation of soil after drainageof the underground water and elimination of pore water pressure inside the soil, and it may take a few months to a few years to reach a stabilized level. In dry soilconditions, the long-term settlements may be considered as very limited.There are mainly three settlement prediction approaches for mechanized tunnel excavations: (1) numerical analysis such as finite element method, (2) analytical method and (3) semi-theoretical (semi-empirical) method. Among them, the numerical approaches are the most reliable ones. However, the results of all methods should be used carefully by an experienced field engineer in designing the stage of an excavation project.In this study, all three prediction methods are employed for a critical zone to predict the short-term maximum surface settlements above the twin tunnels of the chainage between 0 ? 850 and 0 ? 900 m between Esenler and Kirazlıstations of Istanbul Metro line, which is 4 km in length. Plaxis finite element modeling program is used fornumerical modeling; the method suggested by Loganathan and Poulos (1998) is used for the analytical solution. A few different semi-theoretical models are also used for predictions. The results are compared and validated by field measurements. Description of the project, site and construction methodThe first construction phase of Istanbul Metro line was started in 1992 and opened to public in 2000. This line is being extended gradually, as well as new lines are being constructed in other locations. One of these metro lines is the twin line between Esenler and Basaksehir, which is 21.2 km. The excavation of this section has been started in May 2006. Currently, around 1,400 m of excavationhas already been completed. The region is highly populated including several story buildings, industrial zones and heavy traffic. Alignment and stations of the metro line between Esenler and Basaksehir is presented in Fig. 1.Totally four earth pressure balance (EPB) tunnel boring machines (TBM) are used for excavation of the tunnels. The metro lines in the study area are excavated by a Herrenknecht EPB-TBM in the right tube and a Lovat EPB-TBM in the left tube. Right tube excavationfollows around 100 m behind the left tube. Some of the technical features of the machines are summarized in Table 1.Excavated material is removed by auger (screw conveyor) through the machine to a belt conveyor and than loaded to rail cars for transporting to the portal. Since the excavated ground bears water and includes stability problems, the excavation chamber is pressurized by 300 kPa and conditioned by applying water, foam, bentonite and polymers through the injection ports. Chamber pressure is continuously monitored by pressure sensors inside thechamber and auger. Installation of a segment ring with 1.4-m length (inner diameter of 5.7 m and outer diameter of 6.3 m) and 30-cm thickness is realized by a wing-type vacuum erector. The ring is configured as five segments plus a key segment. After installation of the ring, the excavation restarts and the void between the segment outer perimeter and excavated tunnel perimeter is grouted by300 kPa of pressure through the grout cannels in the trailing shield. This method of construction has beenproven to minimize the surface settlements.The study area includes the twin tunnels of the chainage between 0 + 850 and 0 + 900 m, between Esenler and Kirazlı stations. Gungoren Formation of th e Miosen age is found in the study area. Laboratory and in situ tests are applied to define the geotechnical features of theformations that the tunnels pass through. The name, thickness and some of the geotechnical properties of the layers are summarized in Table 2 (Ayson 2005). Fill layer of 2.5-m thick consists of sand, clay, gravel and some pieces of masonry. The very stiff clay layer of 4 m is grayish green in color, consisting of gravel and sand. The dense sand layer of 5 m is brown at the upper levels and greenish yellow at the lower levels, consisting of clay, silt and mica. Dense sand of 3 m is greenish yellow and consists of mica. The base layer of the tunnel is hard clay, which is dark green, consisting of shell. The underground water table starts at 4.5 m below the surface. The tunnel axis is 14.5 m below the surface, close to the contact between very dense sand and hard clay. This depth isquite uniform in the chainage between 0 + 850 and 0 + 900 m.Surface settlement prediction with finite element modelingPlaxis finite element code for soil and rock analysis is used to predict the surface settlement. First, the right tube is constructed, and then the left tube 100 m behind the right tube is excavated. This is based on the assumption that ground deformations caused by the excavation of the right tube are stabilized before the excavation of the left tube. The finite element mesh is shown in Fig. 2 using 15 stress point triangular elements. The FEM model consists of 1,838 elements and 15,121 nodes. In FE modeling, the Mohr–Coulomb failure criterion is applied.Staged construction is used in the FE model. Excavation of the soil and the construction of the tunnel lining are carried out in different phases. In the first phase, the soil in front of TBM is excavated, and a support pressure of 300 kPa is applied at the tunnel face to prevent failure at the face. In the first phase, TBM is modeled as shell elements. In the second phase, the tunnel lining is constructedusing prefabricated concrete ring segments, which are bolted together within the tunnel boring machine. During the erection of the lining, TBM remains stationary. Once a lining ring has been bolted, excavation is resumed until sufficient soil excavation is carried out for the next lining. The tunnel lining is modeled using volume elements. In the second phase, the lining is activated and TBM shell elements are deactivated.When applying finite element models, volume loss values are usually assumed prior to excavation. In this study, the FEM model is run with the assumption of 0.5, 0.75, 1 and 1.5% volume loss caused by the convergence of the ground into the tunnel after excavation. Figures 3 and 4 show total and vertical deformations after both tubes are constructed. The vertical ground settlement profile after theright tube construction is given in Fig. 5, which is in theshape of a Gaussian curve, and that after construction of both tubes is given in Fig. 6. Figure 7 shows the total deformation vectors.The maximum ground deformations under different volume loss assumptions are summarized in Table 3.Surface settlement prediction with semi-theoretical and analytical methodsSemi-theoretical predictions for short-term maximum settlement are performed using the Gaussian curve approach, which is a classical and conventional method. The settlement parameters used in semi-theoretical estimations and notations are presented in Fig. 8.The theoretical settlement (Gaussian) curve is presented as in Eq. 1 (O’Reilly and New 1982):)2(m a x 22i x e S S -= (1)where, S is the theoretical settlement (Gauss error function, normal probability curve), Smax is the maximum short-term (initial, undrained) settlement at the tunnel centerline (m), x is the transverse horizontal distance from the tunnel center line (m), and i is the point of inflexion (m). To determine the shape of a settlement curve, it is necessary to predict i and Smax values.There are several suggested methods for prediction of the point of inflexion (i). Estimation of i value in this studyis based on averages of some empirical approaches given in Eqs. 2–6:where, Z0 is the tunnel axis depth (m), 14.5 m in this study, and R is the radius of tunnel, 3.25 m in this study. Equation 3 was suggested by Glossop (O’Reilly and New 1982) for mostly cohesive grounds; Eq. 4 was suggested by O’Reilly and New (1982) for excavation of cohesive grounds by shielded machines; Eq. 5 was suggested by Schmidt (1969) for excavation of clays by shielded machines; Eq. 6 was suggested by Arioglu (1992) for excavation of all types of soils by shielded machines. As a result, the average i value is estimated to be 6.6 m in this study.There are several suggested empirical methods for the prediction of the maximum surface settlement (Smax).Schmidt suggested a model for the estimation of Smax value for a single tunnel in 1969 as given in Eq. 7 (through Arioglu 1992):where, K is the volume loss (%). Arioglu (1992), based on field data, found a good relationship between K and N (stability ratio) for face-pressurized TBM cases as in Eq. 8:where cn is the natural unit weight of the soil (kN/m3), the weighted averages for all the layers, which is 19 kN/m3 in this study; rS is the total surcharge pressure (kPa), assumed to be 20 kPa in this study; rT is TBM face pressure (kPa), which is 300 kPa in this study; and CU is the undrained cohesion of the soil (kPa), the weightedaverages for all the layers, which is 50 kPa in this study assuming that CU is equal to SU (undrained shear strength of the soil). Allaverages are estimated up to very dense sand, excluding hard clay, since the tunnel axis passes around the contact between very dense sand and hard clay. The model yields 17.1 mm of initial maximum surface settlement.Herzog suggested a model for the estimation of Smax value in 1985 as given in Eq. 9 for a single tunnel and Eq. 10 for twin tunnels (through Arioglu 1992):where, E is the elasticity modulus of formation (kPa), the weighted averages for all the layers, which is 30,000 kPa in this study, and a is the distance between the tunnel axes, which is 14 m in this study. The model yields 49.9 and 58.7 mm of initial maximum surface settlements for the right and the left tube tunnel, which is 100 mm behind the right tube, respectively.There are several analytical models for the prediction of short-term maximum surface settlements for shielded tunneling operations (Lee et al. 1992; Loganathan and Poulos 1998; Chi et al. 2001; Chou and Bobet 2002; Park 2004). The method suggested by Loganathan and Poulos (1998) is used in this study. In this method, a theoretical gapparameter (g) is defined based on physical gap in the void, face losses and workmanship value, and then the gap parameter is incorporated to a closed form solution to predict elastoplastic ground deformations. The undrained gap parameter (g) is estimated by Eq. 12:where Gp is the physical gap representing the geometric clearance between the outer skin of the shield and the liner, is the thickness of the tail shield, d is the clearance required for erection of the liner, U*3D is the equivalent 3D elastoplastic deformation at the tunnel face, and w is a value that takes into account the quality of workmanship.Maximum short-term surface settlement is predicted by theoretical Eq. 13 (Loganathan and Poulos 1998):where, t is undrained Poisson’s ratio, assumed to be of maximum 0.5; g is the gap parameter (m), which is estimated to be 0.0128 m in this study; and x is transversedistance from the tunnel centerline (m) and it is assumed to be 0 m for the maximum surface settlement. The model yields 23.0 mm of undrained maximum surface settlement.Other parameters of settlement such as maximum slope, maximum curvature and so on are not mentioned in this study.Verification of predictions by field measurements and discussionThe results of measurements performed on the surface monitoring points, by Istanbul Metropolitan Municipality, are presented in Table 4 for the left and right tubes. As seen, the average maximum surface settlements are around 9.6 mm for the right tube and 14.4 mm for the left tube, which excavates 100 m behind the right tube. Themaximum surface settlements measured around 15.2 mm for the right tube and 26.3 mm for the left tube. Higher settlements are expected in the left tube since the previous TBM excavation activities on the right tube overlaps the previous deformation. The effect of the left tube excavation on deformations of the right tube is presented in Fig. 9. As seen, after Lovat TBM in the right tube excavates nearby the surface monitoring point 25, maximum surface settlement reaches at around 9 mm; however, while Herrenknecht TBM in the left tube passes the same point, maximum surface settlement reaches at around 29 mm (Fig. 10).If the construction method applied to the site is considered, long-term (consolidation) settlements are expected to be low, since the tail void is grouted immediately after excavation. The results of predictions mentioned above and observed maximum surface settlements are summarized in Table 5.The methods suggested by Loganathan and Poulos (1998) and Schmidt (1969) connected with Arioglu’s suggestion (1992) can predict the maximum short-term surface settlements only for a single tunnel. Plaxis finite element and Herzog (1985) models can predict deformations for twin tubes.Herzog’s model (1985) yields higher maximum surface settlements than the observed ones. The reason for that is that the database of the model includes both shielded tunnels and NATM (New Austrian Tunneling Method) tunnels, of which surfacesettlements are usually higher compared to shielded tunnels. Schmidt (1969), along withArioglu’s suggestion (1992), yields predictions close to observed.Plaxis finite element modeling gives the most realistic results, provided there is correct assumption of volume loss parameter, which is usually difficult to predict. The model provides simulation of excavation, lining, grouting and face pressure in a realistic manner to predict surface and sub-surface settlements. The volume loss parameter is usually assumed to be \1% for excavation with facepressure-balanced tunnel boring machines. The realized volume loss in the site is around 1% for this study.Currently, there is difficulty yet in modeling the deformation behavior of twin tunnels. One of the most impressive studies on this issue was performed by Chapman et al. (2004). However, Chapman’s semi-theoretical method still requires enlargement of the database to improve the suggested model in his paper.ConclusionsIn this study, three surface settlement prediction methods for mechanized twin tunnel excavations be tween Esenler and Kirazlı stations of Istanbul Metro Line are applied. Tunnels of 6.5-m diameters with 14-m distance between their centers are excavated by EPM tunnel boring machines. The geologic structure of the area can be classified as soft ground.Settlement predictions are performed by using FE modeling, and semi-theoretical (semi-empirical) and analytical methods. The measured results after tunneling are compared to predicted results. These indicate that the FE model predicts well the short time surface settlements for a given volume loss value. The results of some semi-theoretical and analytical methods are found to be in goodagreement with the FE model, whereas some methods overestimate the measured settlements. The FE model predicted the maximum surface settlement as 15.89 mm (1% volume loss) for the right tube, while the measured maximum settlement was 15.20 mm. For the left tube (opened after the right), FE prediction was 24.34 mm, while measured maximum settlement was 26.30 mm.中文翻译基于盾构法的Istanbul地铁施工引起的地面沉降预测摘要在这项研究中,研究的是双线隧道的短期地面沉降,选取线路里程总长为4km的Istanbul地铁从Esenler站到Kirazl站方向850到900m区间为研究对象。
建筑防火中英文对照外文翻译文献
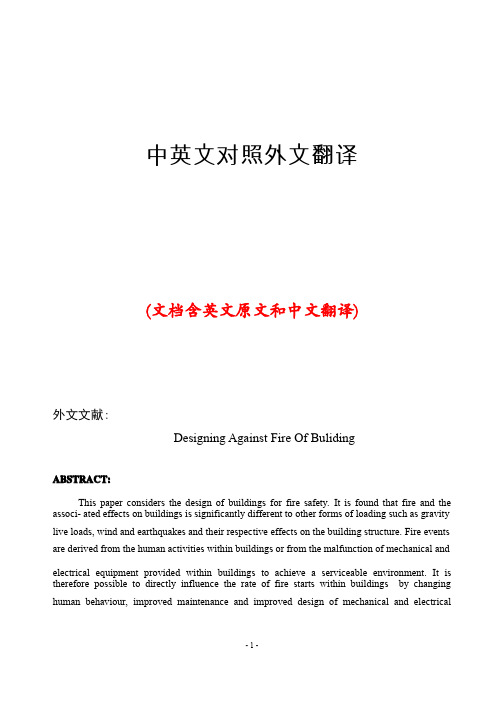
- 1 -中英文对照外文翻译(文档含英文原文和中文翻译)外文文献外文文献: :Designing Against Fire Of BulidingABSTRACT:This paper considers the design of buildings for fire safety. It is found that fire and the associ- ated effects on buildings is significantly different to other forms of loading such as gravity live loads, wind and earthquakes and their respective effects on the building structure. Fire events are derived from the human activities within buildings or from the malfunction of mechanical and electrical equipment provided within buildings to achieve a serviceable environment. It is therefore possible to directly influence the rate of fire starts within buildings by changing human behaviour, improved maintenance and improved design of mechanical and electricalsystems. Furthermore, should a fire develops, it is possible to directly influence the resulting fire severity by the incorporation of fire safety systems such as sprinklers and to provide measures within the building to enable safer egress from the building. The ability to influence the rate of fire starts and the resulting fire severity is unique to the consideration of fire within buildings since other loads such as wind and earthquakes are directly a function of nature. The possible approaches for designing a building for fire safety are presented using an example of a multi-storey building constructed over a railway line. The design of both the transfer structure supporting the building over the railway and the levels above the transfer structure are consideredin the context of current regulatory requirements. The principles and assumptions associ- ated with various approaches are discussed.1 INTRODUCTIONOther papers presented in this series consider the design of buildings for gravity loads, wind and earthquakes.The design of buildings against such load effects is to a large extent covered by engineering based standards referenced by the building regulations. This is not the case, to nearly the same extent, in the case of fire. Rather, it is building regulations such as the Building Code of Australia (BCA) that directly specify most of the requirements for fire safety of buildings with reference being made to Standards such as AS3600 or AS4100 for methods for determining the fire resistance of structural elements.The purpose of this paper is to consider the design of buildings for fire safety from an engineering perspective (as is currently done for other loads such as wind or earthquakes), whilst at the same time,putting such approaches in the context of the current regulatory requirements.At the outset,it needs to be noted that designing a building for fire safety is far more than simply considering the building structure and whether it has sufficient structural adequacy.This is because fires can have a direct influence on occupants via smoke and heat and can grow in size and severity unlike other effects imposed on the building. Notwithstanding these comments, the focus of this paper will be largely on design issues associated with the building structure.Two situations associated with a building are used for the purpose of discussion. The multi-storey office building shown in Figure 1 is supported by a transfer structure that spans over a set of railway tracks. It is assumed that a wide range of rail traffic utilises these tracks including freight and diesel locomotives. The first situation to be considered from a fire safety perspective is the transfer structure.This is termed Situation 1 and the key questions are: what level of fire resistance is required for this transfer structure and how can this be determined? This situation has been chosen since it clearly falls outside the normal regulatory scope of most build-ing regulations. An engineering solution, rather than a prescriptive one is required. The second fire situation (termed Situation 2) corresponds to a fire within the office levels of the building and is covered by building regulations. This situation is chosen because it will enable a discussion of engineering approaches and how these interface with the building regulations regulations––since both engineering and prescriptive solutions are possible.2 UNIQUENESS OF FIRE2.1 Introduction Wind and earthquakes can be considered to b Wind and earthquakes can be considered to be “natural” phenomena o e “natural” phenomena o e “natural” phenomena over which designers ver which designers have no control except perhaps to choose the location of buildings more carefully on the basis of historical records and to design building to resist sufficiently high loads or accelerations for the particular location. Dead and live loads in buildings are the result of gravity. All of these loads are variable and it is possible (although generally unlikely) that the loads may exceed the resistance of the critical structural members resulting in structural failure.The nature and influence of fires in buildings are quite different to those associated with other“loads” to which a building may be subjected to. The essential differences are described in the following sections.2.2 Origin of FireIn most situations (ignoring bush fires), fire originates from human activities within the building or the malfunction of equipment placed within the building to provide a serviceable environment. It follows therefore that it is possible to influence the rate of fire starts by influencing human behaviour, limiting and monitoring human behaviour and improving the design of equipment and its maintenance. This is not the case for the usual loads applied to a building.2.3 Ability to InfluenceSince wind and earthquake are directly functions of nature, it is not possible to influence such events to any extent. One has to anticipate them and design accordingly. It may be possibleto influence the level of live load in a building by conducting audits and placing restrictions on contents. However, in the case of a fire start, there are many factors that can be brought to bear to influence the ultimate size of the fire and its effect within the building. It is known that occupants within a building will often detect a fire and deal with it before it reaches a sig- nificant size. It is estimated that less than one fire in five (Favre, 1996) results in a call to the fire brigade and for fires reported to the fire brigade, the majority will be limited to the room of fire origin. Inoc- cupied spaces, olfactory cues (smell) provide powerful evidence of the presence of even a small fire. The addition of a functional smoke detection system will further improve the likelihood of detection and of action being taken by the occupants.Fire fighting equipment, such as extinguishers and hose reels, is generally provided within buildings for the use of occupants and many organisations provide training for staff in respect ofthe use of such equipment.The growth of a fire can also be limited by automatic extinguishing systems such as sprinklers, which can be designed to have high levels of effectiveness.Fires can also be limited by the fire brigade depending on the size and location of the fire at the time of arrival.2.4 Effects of FireThe structural elements in the vicinity of the fire will experience the effects of heat. The temperatures within the structural elements will increase with time of exposure to the fire, the rate of temperature rise being dictated by the thermal resistance of the structural element and the severity of the fire. The increase in temperatures within a member will result in both thermal expansion and,eventually,a reduction in the structural resistance of the member. Differential thermal expansion will lead to bowing of a member. Significant axial expansion willbe accommodated in steel members by either overall or local buckling or yielding of local- ised regions. These effects will be detrimental for columns but for beams forming part of a floorsystem may assist in the development of other load resisting mechanisms (see Section 4.3.5).With the exception of the development of forces due to restraint of thermal expansion, fire does not impose loads on the structure but rather reduces stiffness and strength. Such effects are not instantaneous but are a function of time and this is different to the effects of loads such as earthquake and wind that are more or less instantaneous.Heating effects associated with a fire will not be significant or the rate of loss of capacity will be slowed if:(a) the fire is extinguished (e.g. an effective sprinkler system)(b) the fire is of insufficient severity –– insufficient fuel, and/or(b) the fire is of insufficient severity(c)the structural elements have sufficient thermal mass and/or insulation to slow the rise in internal temperatureFire protection measures such as providing sufficient axis distance and dimensions for concrete elements, and sufficient insulation thickness for steel elements are examples of (c). These are illustrated in Figure 2.The two situations described in the introduction are now considered.3 FIRE WITHIN BUILDINGS3.1 Fire Safety ConsiderationsThe implications of fire within the occupied parts of the office building (Figure 1) (Situation 2) are now considered. Fire statistics for office buildings show that about one fatality is expected in an office building for every 1000 fires reported to the fire brigade. This is an orderof magnitude less than the fatality rate associated with apartment buildings. More than two thirdsof fires occur during occupied hours and this is due to the greater human activity and the greater use of services within the building. It is twice as likely that a fire that commences out of normal working hours will extend beyond the enclosure of fire origin.A relatively small fire can generate large quantities of smoke within the floor of fire origin.If the floor is of open-plan construction with few partitions, the presence of a fire during normal occupied hours is almost certain to be detected through the observation of smoke on the floor. The presence of full height partitions across the floor will slow the spread of smoke and possibly also the speed at which the occupants detect the fire. Any measures aimed at improving housekeeping, fire awareness and fire response will be beneficial in reducing the likelihood of major fires during occupied hours.For multi-storey buildings, smoke detection systems and alarms are often provided to give “automatic” detection and warning to the occupants. An alarm signal is also transm itted to the fire brigade.Should the fire not be able to be controlled by the occupants on the fire floor, they will need to leave the floor of fire origin via the stairs. Stair enclosures may be designed to be fire-resistant but this may not be sufficient to keep the smoke out of the stairs. Many buildings incorporate stair pressurisation systems whereby positive airflow is introduced into the stairs upon detection of smoke within the building. However, this increases the forces required to open the stair doors and makes it increasingly difficult to access the stairs. It is quite likely that excessive door opening forces will exist(Fazio et al,2006)From a fire perspective, it is common to consider that a building consists of enclosures formed by the presence of walls and floors.An enclosure that has sufficiently fire-resistant boundaries (i.e. walls and floors) is considered to constitute a fire compartment and to be capableof limiting the spread of fire to an adjacent compartment. However, the ability of such boundariesto restrict the spread of fire can be severely limited by the need to provide natural lighting (windows)and access openings between the adjacent compartments (doors and stairs). Fire spread via the external openings (windows) is a distinct possibility given a fully developed fire. Limit- ing the window sizes and geometry can reduce but not eliminate the possibility of vertical fire spread.By far the most effective measure in limiting fire spread, other than the presence of occupants, is an effective sprinkler system that delivers water to a growing fire rapidly reducing the heat being generated and virtually extinguishing it.3.2 Estimating Fire SeverityIn the absence of measures to extinguish developing fires, or should such systems fail; severe fires can develop within buildings.In fire engineering literature, the term “fire load” refers to the quantity of combustibles within an enclosure and not the loads (forces) applied to the structure during a fire. Similarly, fire load density refers to the quantity of fuel per unit area. It is normally expressed in terms of MJ/m2or kg/m 2of wood equivalent. Surveys of combustibles for various occupancies (i.e offices, retail,hospitals, warehouses, etc)have been undertaken and a good summary of the available data is given in FCRC (1999). As would be expected, the fire load density is highly variable. Publications such as the International Fire Engineering Guidelines (2005) give fire load data in terms of the mean and 80th percentile.The latter level of fire load density is sometimes taken asthe characteristic fire load density and is sometimes taken as being distributed according to a Gumbel distribution (Schleich et al, 1999).The rate at which heat is released within an enclosure is termed the heat release rate (HRR) and normally expressed in megawatts (MW). The application of sufficient heat to a combustible material results in the generation of gases some of which are combustible. This process is called pyrolisation.Upon coming into contact with sufficient oxygen these gases ignite generating heat. The rate of burning(and therefore of heat generation) is therefore dependent on the flow of air to the gases generated by the pyrolising fuel.This flow is influenced by the shape of the enclosure (aspect ratio), and the position and size of any potential openings. It is found from experiments with single openings in approximately cubic enclosures that the rate of burning is directly proportional to A h where A is the area of the opening and h is the opening height. It is known that for deep enclosures with single openings that burning will occur initially closest to the opening moving back into the enclosure once the fuel closest to the opening is consumed (Thomas et al, 2005). Significant temperature variations throughout such enclosures can be expected.The use of the word ‘opening’ in relation to real building enclosures refers to any openings present around the walls including doors that are left open and any windows containing non fire-resistant glass.It is presumed that such glass breaks in the event of development of a significant fire. If the windows could be prevented from breaking and other sources of air to the enclosure limited, then the fire would be prevented from becoming a severe fire.V arious methods have been developed for determining the potential severity of a fire within an enclosure.These are described in SFPE (2004). The predictions of these methods are variable and are mostly based on estimating a representative heat release rate (HRR) and the proportion of total fuel ς likely to be consumed during the primary burning stage (Figure 4). Further studies of enclosure fires are required to assist with the development of improved models,as the behaviour is very complex.3.3 Role of the Building StructureIf the design objectives are to provide an adequate level of safety for the occupants and protection of adjacent properties from damage, then the structural adequacy of the building in fire need only be sufficient to allow the occupants to exit the building and for the building to ultimately deform in a way that does not lead to damage or fire spread to a building located on an adjacent site.These objectives are those associated with most building regulations including the Building Code of Australia (BCA). There could be other objectives including protection of the building against significant damage. In considering these various objectives, the following should be taken into account when considering the fire resistance of the building structure.3.3.1 Non-Structural ConsequencesSince fire can produce smoke and flame, it is important to ask whether these outcomes will threaten life safety within other parts of the building before the building is compromised by a lossof structural adequacy? Is search and rescue by the fire brigade not feasible given the likely extent of smoke? Will the loss of use of the building due to a severe fire result in major property and income loss? If the answer to these questions is in the affirmative, then it may be necessary to minimise the occurrence of a significant fire rather than simply assuming that the building structure needs to be designed for high levels of fire resistance. A low-rise shopping centre with levels interconnected by large voids is an example of such a situation.3.3.2 Other Fire Safety SystemsThe presence of other systems (e.g. sprinklers) within the building to minimise the occurrence of a serious fire can greatly reduce the need for the structural elements to have high levels of fire resistance. In this regard, the uncertainties of all fire-safety systems need to be considered. Irrespective of whether the fire safety system is the sprinkler system, stair pressurisation, compartmentation or the system giving the structure a fire-resistance level (e.g. concrete cover), there is an uncertainty of performance. Uncertainty data is available for sprinkler systems(because it is relatively easy to collect) but is not readily available for the other fire safety systems. This sometimes results in the designers and building regulators considering that only sprinkler systems are subject to uncertainty. In reality, it would appear that sprinklers systems have a high level of performance and can be designed to have very high levels of reliability.3.3.3 Height of BuildingIt takes longer for a tall building to be evacuated than a short building and therefore the structure of a tall building may need to have a higher level of fire resistance. The implications of collapse of tall buildings on adjacent properties are also greater than for buildings of only several storeys.3.3.4 Limited Extent of BurningIf the likely extent of burning is small in comparison with the plan area of the building, then the fire cannot have a significant impact on the overall stability of the building structure. Examples of situations where this is the case are open-deck carparks and very large area building such as shopping complexes where the fire-effected part is likely to be small in relation to area of the building floor plan.3.3.5 Behaviour of Floor ElementsThe effect of real fires on composite and concrete floors continues to be a subject of much research.Experimental testing at Cardington demonstrated that when parts of a composite floor are subject to heating, large displacement behaviour can develop that greatly assists the load carrying capacity of the floor beyond that which would predicted by considering only the behaviour of the beams and slabs in isolation.These situations have been analysed by both yield line methods that take into account the effects of membrane forces (Bailey, 2004) and finite element techniques. In essence, the methods illustrate that it is not necessary to insulate all structural steel elements in a composite floor to achieve high levels of fire resistance.This work also demonstrated that exposure of a composite floor having unprotected steel beams, to a localised fire, will not result in failure of the floor.A similar real fire test on a multistory reinforced concrete building demonstrated that the real structural behaviour in fire was significantly different to that expected using small displacement theory as for normal tempera- ture design (Bailey, 2002) with the performance being superior than that predicted by considering isolated member behaviour.3.4 Prescriptive Approach to DesignThe building regulations of most countries provide prescriptive requirements for the design of buildings for fire.These requirements are generally not subject to interpretation and compliance with them makes for simpler design approvalapproval––although not necessarily the most cost-effective designs.These provisions are often termed deemed-to-satisfy (DTS) provisions. Allcovered––the provision of emergency exits, aspects of designing buildings for fire safety are coveredspacings between buildings, occupant fire fighting measures, detection and alarms, measures for automatic fire suppression, air and smoke handling requirements and last, but not least, requirements for compartmentation and fire resistance levels for structural members. However, there is little evidence that the requirements have been developed from a systematic evaluation of fire safety. Rather it would appear that many of the requirements have been added one to anotherto deal with another fire incident or to incorporate a new form of technology. There does not appear to have been any real attempt to determine which provision have the most significant influence on fire safety and whether some of the former provisions could be modified.The FRL requirements specified in the DTS provisions are traditionally considered to result in member resistances that will only rarely experience failure in the event of a fire.This is why it is acceptable to use the above arbitrary point in time load combination for assessing members in fire. There have been attempts to evaluate the various deemed-to-satisfy provisions (particularly the fire- resistance requirements)from a fire-engineering perspective taking into account the possible variations in enclosure geometry, opening sizes and fire load (see FCRC, 1999).One of the outcomes of this evaluation was the recognition that deemed-to- satisfy provisions necessarily cover the broad range of buildings and thus must, on average, be quite onerous because of the magnitude of the above variations.It should be noted that the DTS provisions assume that compartmentation works and that fire is limited to a single compartment. This means that fire is normally only considered to exist at one level. Thus floors are assumed to be heated from below and columns only over one storey height.3.5 Performance-Based DesignAn approach that offers substantial benefits for individual buildings is the move towards performance-based regulations. This is permitted by regulations such as the BCA which state thata designer must demonstrate that the particular building will achieve the relevant performance requirements. The prescriptive provisions (i.e. the DTS provisions) are presumed to achieve these requirements. It is necessary to show that any building that does not conform to the DTS provisions will achieve the performance requirements.But what are the performance requirements? Most often the specified performance is simplya set of performance statements (such as with the Building Code of Australia)with no quantitative level given. Therefore, although these statements remind the designer of the key elements of design, they do not, in themselves, provide any measure against which to determine whether the design is adequately safe.Possible acceptance criteria are now considered.3.5.1 Acceptance CriteriaSome guidance as to the basis for acceptable designs is given in regulations such as the BCA. These and other possible bases are now considered in principle.(i)compare the levels of safety (with respect to achieving each of the design objectives) of the proposed alternative solution with those asso- ciated with a corresponding DTS solution for the building.This comparison may be done on either a qualitative or qualitative risk basis or perhaps a combination. In this case, the basis for comparison is an acceptable DTS solution. Such an approach requires a “holistic” approach to safety whereby all aspects relevant to safety, including the structure, are considered. This is, by far, the most common basis for acceptance.(ii)undertake a probabilistic risk assessment and show that the risk associated with the proposed design is less than that associated with common societal activities such as using pub lic transport. Undertaking a full probabilistic risk assessment can be very difficult for all but the simplest situations.Assuming that such an assessment is undertaken it will be necessary for the stakeholders to accept the nominated level of acceptable risk. Again, this requires a “holistic” approach to fire safety.(iii) a design is presented where it is demonstrated that all reasonable measures have been adopted to manage the risks and that any possible measures that have not been adopted will have negligible effect on the risk of not achieving the design objectives.(iv) as far as the building structure is concerned,benchmark the acceptable probability of failure in fire against that for normal temperature design. This is similar to the approach used when considering Building Situation 1 but only considers the building structure and not the effects of flame or smoke spread. It is not a holistic approach to fire safety.Finally, the questions of arson and terrorism must be considered. Deliberate acts of fire initiation range from relatively minor incidents to acts of mass destruction.Acts of arson are well within the accepted range of fire events experienced by build- ings(e.g. 8% of fire starts in offices are deemed "suspicious"). The simplest act is to use a small heat source to start a fire. The resulting fire will develop slowly in one location within the building and will most probably be controlled by the various fire- safety systems within the building. The outcome is likely to be the same even if an accelerant is used to assist fire spread.An important illustration of this occurred during the race riots in Los Angeles in 1992 (Hart 1992) when fires were started in many buildings often at multiple locations. In the case of buildings with sprinkler systems,the damage was limited and the fires significantly controlled.Although the intent was to destroy the buildings,the fire-safety systems were able to limit the resulting fires. Security measures are provided with systems such as sprinkler systems and include:- locking of valves- anti-tamper monitoring- location of valves in secure locationsFurthermore, access to significant buildings is often restricted by security measures.The very fact that the above steps have been taken demonstrates that acts of destruction within buildings are considered although most acts of arson do not involve any attempt to disable the fire-safety systems.At the one end of the spectrum is "simple" arson and at the other end, extremely rare acts where attempts are made to destroy the fire-safety systems along with substantial parts of thebuilding.This can be only achieved through massive impact or the use of explosives. The latter may be achieved through explosives being introduced into the building or from outside by missile attack.The former could result from missile attack or from the collision of a large aircraft. The greater the destructiveness of the act,the greater the means and knowledge required. Conversely, the more extreme the act, the less confidence there can be in designing against such an act. This is because the more extreme the event, the harder it is to predict precisely and the less understood will be its effects. The important point to recognise is that if sufficient means can be assembled, then it will always be possible to overcome a particular building design.Thus these acts are completely different to the other loadings to which a building is subjected such as wind,earthquake and gravity loading. This is because such acts of destruction are the work of intelligent beings and take into account the characteristics of the target.Should high-rise buildings be designed for given terrorist activities,then terrorists will simply use greater means to achieve the end result.For example, if buildings were designed to resist the impact effects from a certain size aircraft, then the use of a larger aircraft or more than one aircraft could still achieve destruction of the building. An appropriate strategy is therefore to minimise the likelihood of means of mass destruction getting into the hands of persons intent on such acts. This is not an engineering solution associated with the building structure.It should not be assumed that structural solutions are always the most appropriate, or indeed, possible.In the same way, aircrafts are not designed to survive a major fire or a crash landing but steps are taken to minimise the likelihood of either occurrence.The mobilization of large quantities of fire load (the normal combustibles on the floors) simultaneously on numerous levels throughout a building is well outside fire situations envisaged by current fire test standards and prescriptive regulations. Risk management measures to avoid such a possibility must be considered.4 CONCLUSIONSificantly from other “loads” such as wind, live load and earthquakes in significantlyFire differs signrespect of its origin and its effects.Due to the fact that fire originates from human activities or equipment installed within buildings, it is possible to directly influence the potential effects on the building by reducing the rate of fire starts and providing measures to directly limit fire severity.The design of buildings for fire safety is mostly achieved by following the prescriptive requirements of building codes such as the BCA. For situations that fall outside of the scope of such regulations, or where proposed designs are not in accordance with the prescriptive requirements, it is possible to undertake performance-based fire engineering designs.However,。
GMS地面沉降SUB模块例题中文翻译(笔记)

2简介SUB(the subsidence and aquifer-system compaction package)是由美国地质调查局开发的用来模拟含水层压缩和地面沉降的程序包。
Sub程序包模拟包括弹性和非弹性夹层的压缩,也能够模拟夹层的滞后和非滞后排水。
2.1概要下面是操作步骤:1、把sub程序包加入到网格法模拟模型中去2、创建一个简单的概念模型去说明sub程序模块是怎样被概化并且插入到modflow中去的3问题描述我们将要解决的问题将在图1中说明,模型基于美国地质调查局模型,描述如下:羚羊峡谷(antelope)是莫哈维沙漠(mojave)西部的地形封闭的盆地,距离洛杉矶大约50英里。
羚羊峡谷地下水流域面积大约940平方英里,北部被低矮山丘和断层分开,1972年以前,地下水占总供水源的90%多;自1972年以来,供应量占50-90%。
包括正在迅速的发展的城市lancaster和palmdale在内,在羚羊峡谷大规模抽取地下水。
本区地下水系统由上层、中层和下层含水层组成。
含水层由砾石、砂、淤泥和粘土冲积沉积和粘土及淤泥质粘土湖相沉积,为非固结沉积。
研究区之前的地下水开发,补给来自周围山区的径流入渗。
地下水从补给区流向沙漠盆地通过蒸发和泉流出露进行排泄,部分地下水流水平向阻隔,例如断层,已经在地下水流区域确定。
地下水位下降由于地下水开发已经减少了自然因素的排泄,而农村和城市的抽水已经成为了该区地下水系统的主要排泄因素。
回水入渗已经成为了该区地下水系统重要的补给来源。
该模型被离散为网格模型,为43行,60列,3层。
每层对应相应含水层。
模拟期从1915年-1995年80年,并且第一年为稳定状态。
4开始如果必要,打开gms。
如果gms已经运行,选择File | New命令确保项目设置是默认状态5读取工程首先,我们读取工程:1、首先File | Open2、浏览所建工程文件夹3、打开start.gpr.你会看到图1的modflow模型6用网格法加入sub程序包6.1重命名并保存模型我们将要做一下变更,接下来是重命名并且保存模型1、选择file→save as2、确保一直在工程文件夹中3、对该工程重命名为avgrid.gpr.4、保存6.2激活sub程序包我们需要打开sub程序包1、选择MODFLOW | Global Options打开MODFLOW Global/Basic Package对话框2、选择Packages按钮打开MODFLOW Packages对话框3、在Optional packages.下勾选SUB - Subsidence程序包4、确认两次退出对话框6.3定义非滞后夹层总结:定义夹层的前期固结水头、弹性与非弹性储水系数,其中前期固结水头为含水层中初始水头(也即是那个2d散点)首先我们先为第一和第二模型层加入非滞后夹层1、选择MODFLOW | Optional Packages | SUB - Subsidence打开MODFLOW SUB Package对话框这个步骤是打开SUB package对话框。
地下铁道施工引起的地表沉降与变形测量英文版
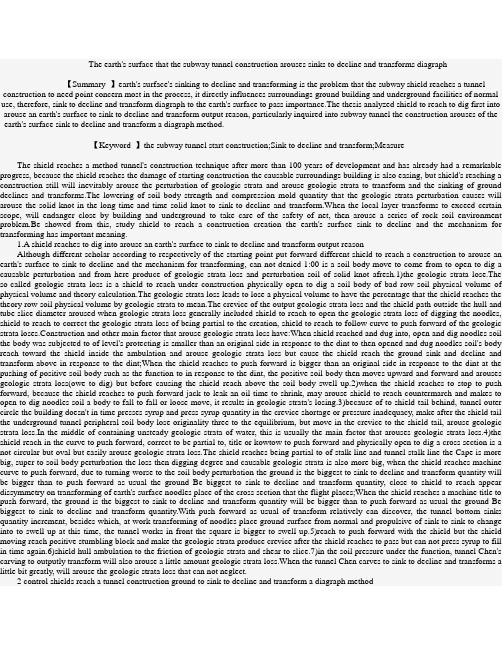
The earth's surface that the subway tunnel construction arouses sinks to decline and transforms diagraph【Summary 】earth's surface's sinking to decline and transforming is the problem that the subway shield reaches a tunnel construction to need point concern most in the process, it directly influences surroundings ground building and underground facilities of normal use, therefore, sink to decline and transform diagraph to the earth's surface to pass importance.The thesis analyzed shield to reach to dig first into arouse an earth's surface to sink to decline and transform output reason, particularly inquired into subway tunnel the construction arouses of the earth's surface sink to decline and transform a diagraph method.【Keyword 】the subway tunnel start construction;Sink to decline and transform;MeasureThe shield reaches a method tunnel's construction technique after more than 100 years of development and has already had a remarkable progress, because the shield reaches the damage of starting construction the causable surroundings building is also easing, but shield's reaching a construction still will inevitably arouse the perturbation of geologic strata and arouse geologic strata to transform and the sinking of ground declines and transforms.The lowering of soil body strength and compression mold quantity that the geologic strata perturbation causes will arouse the solid knot in the long time and time solid knot to sink to decline and transform.When the local layer transforms to exceed certain scope, will endanger close by building and underground to take care of the safety of net, then arouse a series of rock soil environment problem.Be showed from this, study shield to reach a construction creation the earth's surface sink to decline and the mechanism for transforming has important meaning.1.A shield reaches to dig into arouse an earth's surface to sink to decline and transform output reason Although different scholar according to respectively of the starting point put forward different shield to reach a construction to arouse an earth's surface to sink to decline and the mechanism for transforming, can not denied 1:00 is a soil body move to come from to open to dig a causable perturbation and from here produce of geologic strata loss and perturbation soil of solid knot afresh.1)the geologic strata lose.The so-called geologic strata loss is a shield to reach under construction physically open to dig a soil body of bad row soil physical volume of physical volume and theory calculation.The geologic strata loss leads to lose a physical volume to have the percentage that the shield reaches the theory row soil physical volume by geologic strata to mean.The crevice of the output geologic strata loss and the shield path outside the hull and tube slice diameter aroused when geologic strata loss generally included shield to reach to open the geologic strata loss of digging the noodles, shield to reach to correct the geologic strata loss of being partial to the creation, shield to reach to follow curve to push forward of the geologic strata loses.Construction and other main factor that arouse geologic strata loss have:When shield reached and dug into, open and dig noodles soil the body was subjected to of level's protecting is smaller than an original side in response to the dint to then opened and dug noodles soil's body reach toward the shield inside the ambulation and arouse geologic strata loss but cause the shield reach the ground sink and decline and transform above in response to the dint;When the shield reaches to push forward is bigger than an original side in response to the dint at the pushing of positive soil body such as the function to in response to the dint, the positive soil body then moves upward and forward and arouses geologic strata loss(owe to dig) but before causing the shield reach above the soil body swell up.2)when the shield reaches to stop to push forward, because the shield reaches to push forward jack to leak an oil time to shrink, may arouse shield to reach countermarch and makes to open to dig noodles soil a body to fall to fall or loose move, it results in geologic strata's losing.3)because of to shield tail behind, tunnel outer circle the building doesn't in time presses syrup and press syrup quantity in the crevice shortage or pressure inadequacy, make after the shield tail the underground tunnel peripheral soil body lose originality three to the equilibrium, but move in the crevice to the shield tail, arouse geologic strata loss.In the middle of containing unsteady geologic strata of water, this is usually the main factor that arouses geologic strata loss.4)the shield reach in the curve to push forward, correct to be partial to, title or kowtow to push forward and physically open to dig a cross section is a not circular but oval but easily arouse geologic strata loss.The shield reaches being partial to of stalk line and tunnel stalk line the Cape is more big, super to soil body perturbation the loss then digging degree and causable geologic strata is also more big, when the shield reaches machine curve to push forward, due to turning worse to the soil body perturbation the ground is the biggest to sink to decline and transform quantity will be bigger than to push forward as usual the ground Be biggest to sink to decline and transform quantity, close to shield to reach appear dissymmetry on transforming of earth's surface noodles place of the cross section that the flight places;When the shield reaches a machine title to push forward, the ground is the biggest to sink to decline and transform quantity will be bigger than to push forward as usual the ground Be biggest to sink to decline and transform quantity.With push forward as usual of transform relatively can discover, the tunnel bottom sinks quantity increment, besides which, at work transforming of noodles place ground surface from normal and propulsive of sink to sink to change into to swell up at this time, the tunnel works in front the square is bigger to swell up.5)reach to push forward with the shield but the shield moving reach positive stumbling block and make the geologic strata produce crevice after the shield reaches to pass but can not press syrup to fill in time again.6)shield hull ambulation to the friction of geologic strata and shear to slice.7)in the soil pressure under the function, tunnel Chen's carving to outputly transform will also arouse a little amount geologic strata loss.When the tunnel Chen carves to sink to decline and transforms a little bit greatly, will arouse the geologic strata loss that can not neglect.2 control shields reach a tunnel construction ground to sink to decline and transform a diagraph methodSinking to decline to prognosticate to press the part can is divided into a surface layer to sink to decline to prognosticate, deep(layering) sink to decline to prognosticate to sink to decline to prognosticate with cross section.After several years of development, sink to decline to prognosticate a method continuously perfect, prognosticate an instrument type also more and more, but prognosticating of dissimilarity method and prognosticate instrument to be applicable to a different engineering geology condition.Currently, sink to decline to monitor an in common use method to have:monitor stake, sink to decline a cup and sink to decline plank, Ci wreath to sink to decline an instrument, water pressure type a section to sink to decline instrument and level to measure inclined instrument etc..Connect down, will do simple introduction to above these methodses.2.1.Nail to bury with wodden post and steel Han in, hold with the level instrument even, can immediately measure sinking of soil body surface to decline quantity.A monitor stake could observe road noodles to sink to decline last 1:00, if wanted to carry on sinking of the whole cross section to decline diagraph, had to lay several monitor stakes.The size of monitor stake that lays in the different position has a different request.This method is the most simple, but can measurese building surface of sinking and declining value, can not test a soil body inner part some sinking of position decline and contain interference towards filling a soil construction.2.2. sink to decline water cup to measure.Monitor roadbed inner part to transform most effectively, one of the most economic meanses sink to decline water cup to measure.Sink to decline water cup exploitation to connect machine principle, reflect internal a certain Gao Cheng of roadbed to shoot roadbed exterior, the past diagraph roadbed exterior should reflect Gao Cheng who shoot the point namely know Gao Cheng whom the roadbed inner part prognosticates a point.Passing the certain time's segment monitor should reflect to shoot some variety of Gao distance, can immediately be a little bit clear the inner part drawing an exit radicle prognosticate the form of point to change process.But these forms' changing process is the foundation data that studies roadbed to transform mechanism and regulation.Its advantage lies in prognosticating method principle simple and plain easily understand, cost cheap.The weakness is to root-canally lay to have higher request, if laying gravamen were agreeable, easily form a bubble to jam a pipe line, test would not carry on;Don't adapt to as well in colder region;The direct range estimation prognosticates water cup to read the liquid noodles engrave one degree value, the accuracy is lower.(private 1 mm)The method is used for the indoor experiment prognosticate a little bit easily, while useding for actual engineering to prognosticate because of the spot condition complications, the weather variety abnormality, physically operate more difficult, generally and less use.2.3 .sink to decline plank.Sink to decline plank to be generally used for prognosticating a soft land radicle to always sink to decline, test foundation noodles sinking of foundation noodles to decline to distribute under the sistuation that the dissimilarity handles a measure, different lotus to carry.Sink to decline plank as to sink to decline one of the method that the dynamic state prognosticates, with its price low, establish method simple, easily prognosticate quilt extensive adoption, replaced some region instrumentations to prognosticate, but accuracy and precision of data are easily subjected to a shadow to.Sink to decline the plank base sinks to decline along with the roadbed, leveling staff pass to connect with each other with base, can measure the base lay the roadbed of place to sink to decline a value.2.4. Ci wreaths sink to decline an instrument.The Ci wreath sinks to decline an instrument[5] is a layering to sink to decline to make use of the most extensive kind in the instrument.The Ci wreath sinks the work principle Ci wreath of declining the instrument to sink to decline instrument uses and spreads a feeling machine according to the electromagnetic induction principle design, the at all point position that the Ci respond to sink to decline wreath(sink to decline a mark) to in advance pass to drill a hole a way to cover up into an underground to treat to measure, be spread a feeling machine to respond wreath through a Ci, produce electromagnetic induction signal to send to ground gauge to suggest, send out a sound and light to report to the police at the same time.(generally is a buzzer to send out signal)Read bore the marking order a rightness in response to the engraving of steel Chinese foot number of degrees value, fored the depth that sinks and declines wreath.Is general each time measured value with last time measure value to mutually reduce for should measure point of sinking and declining quantity.But physically measure medium can choose a bore according to the condition elevation or most the first floor sink to decline to mark position as to monitor basis to order and pass a rightness the corresponding period don't measure result of contrast analysis, can make sure that sinking of each soil layer declines(or swell up) a result.2.5.The PVC tube measures an inclined instrument.PVC tube's measuring an inclined instrument(also call to measure an inclined instrument is a kind of the home position monitor that measurement drills a hole Qing Cape and azimuth angle.The PVC tube sinks to decline an instrument to press the use method different, can is divided into a glide type to measure inclined instrument and fixed type to measure an inclined instrument.The fixed type is the position that will measure an inclined instrument to measure a head fixedly to move in the soil a certain inside the body, use to deliver the signal electric cable derivation ground to carry on prognosticating.Adopt a fixed type to measure an inclined instrument to prognosticate of measure a point, generally used for a movable type to measure an inclined instrument hard attain to prognosticate of measure a point, its expenses Gao, measure a damage hard maintain, currently adoption very few.The movable type measures an inclined instrument to stretch forward easy to maintain, and can carry on ordering to prognosticate in a row more, apply very extensively.If press the sensitive component different then can is divided into a servo acceleration account type, electric resistance contingency slice type and steel Xian type and bad etc. for moving an electric resistance type.Because the servo acceleration account type measures inclined instrument accuracy Gao, long term stability good, now already drive extensive adoption.If measure a direction according to the instrument different then can is divided into to hang to measure inclined instrument(measure a level to move), level to measure an inclined instrument(measure hang to move to) with inclined to measure an inclined instrument.(measure inclined plane of the method move to)3 conclusionsIn fine, the tunnel geology condition in city is generally very complex, all generally is placed in a lax and weak soil layer in, therefore to subway tunnel construction arouse of the earth's surface sink to decline and transform of prognosticate is importance, sink to decline currently and the method of diagraph for transforming is many, should combine actual circumstance to the adoption of a certain method in the actual engineering to make selection an earth's surface to sink to decline and the diagraph method for transforming.。
地铁隧道施工外文文献翻译

(含:英文原文及中文译文)文献出处:Cocheril Y. Study on Construction Technology of Multi-Arch Tunnel Group in Urban Underground Railway[J]. Journal of Communications, 2015, 3(4):22-32.英文原文Study on Construction Technology of Multi-Arch Tunnel Group in UrbanUnderground RailwayY CocherilAbstractIn this paper, the construction method of the multi-arch tunnel group is discussed by using an engineering example of Metro Line 3. In the construction of the subway, the construction technique of changing a multi-arch tunnel into a single-hole tunnel was first proposed. The technical solutions of the single-middle wall and the separated middle-wall structure were compared and selected to meet the requirements of structural safety, construction safety, and economic efficiency. Good technical solutions can provide reference and reference for the design and construction of similar projects in the future. Keywords: multi-arch tunnel group, single middle partition wall, separated middle partition wall, construction technologyBecause of the design requirements of the subway tunnel, a varietyof tunnel structures are required. Among them, a multi-arch tunnel segment consisting of unequal cross-linked arches and triple-arched tunnels is often used for the connection of the main line and the crossover line. This article combines the project example according to the geological conditions of the tunnel, the time limit requirements through comparison and selection of the best construction program that can achieve rapid construction and save construction costs.1 Project OverviewThe return line of Sports West Road Station on Metro Line 3 is a complex type of return line from Sports West Road Station. In the section , a tunnel group with unequal spans with double arches and triple arches was set up. Unequal cross-arch tunnel excavation span of 20.1m excavation height of 10.076m cross-vector ratio of 1:0.5 hole lining after the lining of 5.2m large-hole lining after the span of 11.4m in the wall thickness of 1.6m. The triple-arch tunnel excavation span is 19.9m and the 7.885m cross-vector ratio is 1:0.1. The surrounding rock of the section of the multi-arch tunnel is from top to bottom: artificial backfill, red sand and alluvial sand layer, alluvial-diluvial earth, fluvial-lacustrine sedimentary soil, plastic residual soil, hard plastic-hard residue. Soil, weathered rock formations, strong weathered rock formations, weathered layers, and weathered layers. Tunnels through the formation of more homogeneous rock strength, strong bearing capacity and stability. Thethickness of the vault covering the tunnel is 15.518m, and the thickness of the surrounding rock layer IV is The buried depth of groundwater in the section of the multi-arch tunnel is , mainly Quaternary pore water and fissure water.2 double arch construction planDue to the complex structure of the multi-arch tunnel section, the tunnel section changes greatly. The construction process is complex and the construction is difficult. The construction period is long. Therefore, it is very important to choose a good construction scheme to complete the construction of the multi-arch tunnel section with high quality and efficiency. When selecting a construction plan, the following aspects are mainly considered: 1 Construction safety and construction Safety 2 Construction difficulty 3 Construction cycle 4 Economic benefits. Based on these four principles, the following two construction plans were selected for comparative selection through the research and demonstration of the construction plan.2.1 Single Wall Construction PlanThe main construction steps and measures of this program are as follows: 1 Prevent the construction of the middle wall from timely construction after the completion of the construction of the temporary construction channel, double-arched and triple-arched intermediate wall from the double-arched tunnel on the right line to the return line side. . 2After the construction of the middle wall lining is completed, the CRD construction method for the right line shall be used for the construction of the large-span tunnel of the re-entry line in accordance with the principle of “small first, large, and closed”. (3) When the construction of the triple-arch tunnel on the side of the re-entry line is carried out, the construction of the triple-arch and double-arched middle wall shall be carried out in accordance with the construction method of the middle-wall of the right line. After the completion of the construction of the four-fold line on the side of the middle wall, the construction of the right line will continue. This construction method is applied to the general construction methods of domestic double-arch tunnels in Guangzhou Metro, Nanjing Metro and Beijing Subway, and can safely and smoothly complete the construction of tunnel groups. However, the study of previous engineering examples and construction techniques can reveal that the program still has shortcomings and defects. 1 This scheme is applied frequently in this project. The initial support and the secondary lining of the tunnel within the short 21.11m multi-arch tunnel will convert 4 times.2 The waterproof layer construction, reinforcement engineering, formwork engineering, and concrete pouring involved in the lining of the middle wall and side tunnels all require multiple conversions and a construction period of up to 2 months. After the completion of the lining, the investment of the anti-bias support of the middle wall and theequipment and equipment will lead to higher construction costs and lower economic benefits.2.2 Separated Wall Construction PlanThe main construction steps and measures of this plan are as follows: 1 Change the unequal span double-arch tunnels into two single holes to change the separation-type mid-rise wall first from the right-line single-line tunnel construction. 2 Double-arched tunnels will not be used for middle-liner lining under single-line conditions. 3 The right-sided large-section double-arch tunnel passes through the side wall of the CRD method. For the 4 fold back line, the construction is performed in the reverse order of the right line. Adopting this scheme is actually a comparison between the two single-line construction methods and the previous one. This has the following advantages: 1 Reduce the number of construction processes and speed up the transition of the process. 2 Reduced the difficulty of construction and shortened the construction period. 3 Reduced construction costs and increased economic efficiency.4 The change to a single wall in the middle of the wall has completely solved the waterproofing defects of the double-arch tunnel structure.5 The construction of the middle tunnel of a triple-arch tunnel is equivalent to a large-span tunnel with reserved core rock, which is conducive to the construction of safety double-arch tunnels on both sides.3 Three Arches Construction PlanFrom the right line directly into the triple-arch tunnel, its supporting parameters are based on the original design, and the entire ring is installed. The whole ring is sprayed on the design and the anchor bar at the middle wall is reinforced. The re-entry side is the same as the right-line construction method. It is necessary to remove a longitudinal reinforcement beam at the junction of the tunnel grille. Strictly control the distance between each step of the excavation footage grid is 0.6m/榀. The middle-wall excavation adopts a weak-weak-weakening blasting scheme to conditionally use the static blasting scheme to minimize the disturbance to the middle-wall rock formation and the lining tunnel to ensure construction safety. The secondary lining is performed immediately after the middle wall excavation is completed. After the completion of the construction of the middle wall, the gaps in the middle walls will be backfilled with jack support. Only one side of the construction is completed before the other side of the wall construction. After the completion of the construction of the middle walls on both sides, the secondary lining of the single-hole tunnels on both sides shall be promptly conducted, and then the excavation and lining of the middle rock mass of the triple-arch tunnel shall be carried out. Special attention should be paid to the settlement and convergence deformation of the triple-arch tunnel at the middle of construction.4 Analysis of structural behavior during constructionChanged the cancellation of mid-walls that do not cross double arches into separated walls. There is no similar engineering design and construction experience in domestic urban subway projects, and there is no similar tunnel structure design. Therefore, whether the structure is safe and whether the construction process is changed during the construction process. Safety will be the focus of this program. Using ANSYS finite element general program software to perform numerical simulations on unequal cross-arch tunnels. The strata-structure model was used to analyze the stress and deformation of the tunnel structure (Fig. 1, Fig. 2, Fig. 3). The horizontal direction of the force taken along the direction of the tunnel is limited to 3 times the hole span. The vertical direction is taken upwards to the surface, and the bottom is 3 times the hole span. Element model Elasto-plastic physical tunnel lining with DP stratum material adopts elasticity The beam element simulation beam elements and solid elements are connected using a coupling equation. It can be seen from the data analysis in Table 2 that the large tunnel has a greater impact on the small tunnel during construction. If the necessary reinforcement measures are taken for the small section tunnel and the longitudinal demolition distance of the temporary support is controlled, this scheme is beneficial and feasible.5 Key Construction Technologies and Corresponding MeasuresThe construction of the multi-arch tunnel section needs to be carriedout under strict construction organization and strong technical guarantee measures. The construction of each construction step is a key to successful construction.5.1 Pulling bolts and reinforcing boltsAfter the removal of the single middle wall, the thickness of the middle wall after the excavation is completed is 0.8m. It is very necessary to set the anchor bolt and the reinforcement bolt. For the tension bolt, the length of the Φ22 steel reel bolt is , and the thickn ess of the middle wall is Reinforced anchor rods are installed at the inverting arch and side wall at both sides of the middle wall with a Φ25 hollow grouting anchor spacing of5.2 Grouting Reinforcement in Middle Wall Rock PillarThe thinnest part of the rock mass in the middle wall is 0.15m. After several blasting excavation processes, the surrounding rock around the middle wall loosens its bearing capacity. Therefore, the loose surrounding rock must be grouted in the vaults, walls and inverted arches of the middle wall. The embedded Φ42 steel pipe slurry adopts a cement-water glass double slurry parameter of 1:1 cement slurry and 3045Be. In the two excavations, the grouting pressure of the inflow glass solution of the middle wall is 0.21.0 MPa. After the final excavation of the grouting line,a saturated grouting is performed on the sandwich wall.5.3 Differential Blasting TechnologyAll the tunnel excavations are drilled and blasted. Because the ground buildings in the downtown area of Guangzhou City are dense and the tunnel is blasted at a distance of “0”, the blasting vibration must be controlled within the allowable range in accordance with the blasting scheme for micro-shock blasting in the reserved smooth layer. The blasting measures taken for Grade III and Grade IV surrounding rocks in the strata of a multi-arch tunnel are as follows: (1) Blasting equipment uses emulsion explosives with low seismic velocity. 2 Strictly control the distance between the perforation of per cycle and the distance between the peripheral blastholes of 0.4m to reduce the charge volume and control the smooth blasting effect. 3 Multi-stage detonator detonation in each blasting The non-electrical millimeter detonator is used to asymmetrically detonate the network micro-vibration technology. 4Second excavation is adopted at the middle wall. 1m is reserved for the smooth surface. Grooves are arranged on the side far away from the middle wall. medicine. The use of artificial wind excavation for excavation of partially dug excavation is prohibited. Through the above-mentioned effective measures, the “0” distance excavation of the multi-arch tunnel was smoothly passed without causing damage to the 0.15-m thick middle wall during the secondary blasting of the middle wall.5.4 Assisted Scissor SupportThrough ANSYS simulation analysis In order to ensure the safety ofsmall-section tunnel construction, it is necessary to assist the reinforcement of the small-section tunnel to withstand the transient impact caused by blasting and the bias generated by the load release during excavation of the rock formation. The support material is welded to both ends of the grid pre-embedded steel plate with I20 steel and the spacing of the support arrangement is 0.6m, ie high strength bolts are used on each grid. The layout of the arrangement was extended to 1.2m on each side of the double arch and completed in front of the big end of the excavation. The height and angle of the support arrangement should ensure smooth construction machinery and equipment. Through the construction proof that the setting of the support is necessary and effective, the small section tunnel converges only 5 mm after the auxiliary scissor is added.5.5 Information ConstructionIn order to ensure structural safety and construction safety, real-time monitoring measurement is carried out during the tunnel construction process. The deformation characteristics of supporting structures and surrounding strata are used to predict the corresponding support structure displacements and to verify the rationality of supporting structures to provide a basis for information construction. Monitoring during construction shows that the maximum settlement of a tunnel with a small cross section is 14.6 mm. The maximum settlement of a tunnel with alarge section is 17.2 mm. The maximum convergence of the tunnel is 7.6 mm. The maximum settlement of the ground is 10 mm. The maximum settlement of the arch with a triple hole arch is 22.8 mm.中文译文城市地下铁道连拱隧道群施工技术研究作者Y Cocheril摘要本文利用地铁三号线某一工程实例对连拱隧道群施工工法进行探讨。
城市地铁隧道施工引起的地面沉降

第38卷第2期2008年3月 东南大学学报(自然科学版)JOURNAL O F SOU THEAST UN I V ERS ITY (N atural Science Edition )V ol 138N o 12M ar .2008城市地铁隧道施工引起的地面沉降缪林昌 王 非 吕伟华(东南大学交通学院,南京210096)摘要:分析了城市隧道施工引起地表沉降的原因,主要包括地层损失和在新的应力状态下土层固结与蠕变方面的原因.通常认为地层损失的体积等于隧道地表沉降槽的体积,而忽略了由于隧道施工降水排水和新的应力状态下土层固结引起的沉降变形.以矿山法施工为例,推导了隧道施工在新的状态下的土体内部孔隙水压消散的公式,进而考虑土体的固结引起的沉降变形.研究成果应用到南京地铁Ⅰ号线鼓楼2玄武门段,根据具体地质条件和矿山法施工的实施进行理论计算分析,结果表明同时考虑地层损失和土体固结变形计算的地表沉降与实测结果吻合较好.关键词:地面沉降;隧道施工;固结;地层损失中图分类号:TU 91;U 21 文献标识码:A 文章编号:1001-0505(2008)022*******Ground surface settle ment due to urban tunnel constructi onM iao L inchang W ang Fe i L üW eihua(School of Transportation,Southeast U niversity,N anjing 210096,China )Abstract:The causes of the g round surface settlem ent due to the urban tunnel construction are ana 2lyzed,w hich includes t w o m ain causes,one is the ground lose,the other is the consolidation and creep of soil layers under new stress state .In general,it is assum ed that the volum e of ground lose during the tunnel construction is equal to the trough vo lum e .This v iew neg lects the effect of dew ate 2ring w ater,drainage of soil layers and the consolidation of so il layers under the new stress state due to the tunnel construction .The pore p ressure dissi p ating for m ula during the tunnel construction is p resented based on the m ining m ethod construction,it can be used to calculate the settlem en t of con 2solidation of so il layers during the tunnel construction .The calculation m ethod has been app lied to the engineering case of the G ulou 2X uanw u section of N o .Ⅰline of N anjing subw ay of C hina .The calculation results of the ground settlem ent are in agreem ent w ith the m easured settlem en ts because the effects of the ground lose and consolidation of soil layers under the new stress state are considered si m u ltaneously .Key words:g round settlem ent ;urban tunnel construction;consolidation;ground lose收稿日期:2007209217. 作者简介:缪林昌(1961—),男,博士,教授,博士生导师,L c .m iao @seu .edu .cn .基金项目:国家自然科学基金资助项目(50478072).引文格式:缪林昌,王非,吕伟华.城市地铁隧道施工引起的地面沉降[J ].东南大学学报:自然科学版,2008,38(2):2932297. 由于在地铁施工过程中不可避免地会产生地层的损失、局部降低地下水位和对地层的扰动,这就必然产生不同程度的地面沉降,从而对地铁施工及周边环境的安全产生不利的影响.在隧道施工中,为避免明挖法带来的大面积拆迁问题,最大限度地减少对沿线居民日常生活和出行的影响,常常采用暗挖施工.隧道矿山法施工因其工程造价明显低于盾构法,尤其在特殊地质地段,以及折返线、渡线、联络通道等复杂断面结构的隧道工程中,矿山法已成为难以取代的方法.但在城市地铁隧道矿山法施工中,如何有效地控制工程质量,确保隧道施工及其周围环境的安全,是地铁施工中必须克服的难题.因此,科学合理地确定地表沉降控制指标,以减轻、避免和消除由于地表沉降产生的不利影响是十分必要的.估计地面变形最主要是受地层损失参数的影响.地层损失分为两阶段:一是不排水的地层损失,这主要是盾构刚刚通过时或者隧道刚刚开挖时所出现的地层损失;二是由于土层固结和蠕变产生的地层损失[1].到目前为止,已有不少关于估计地面沉降(槽)的公式,但这些公式大多只考虑不排水的地层损失,然后进行修正,使得计算值尽可能地接近实测值,并没有考虑土层固结等引起的地面变形.出现这一现象的主要原因在于考虑固结后难度增加了很多,因而如何将固结产生的沉降变形考虑在内始终未能得到很好的解决.本文就南京地铁南北线一期工程鼓楼站2玄武门站区间(鼓2玄区间)隧道采用矿山法施工对周边环境影响进行分析与计算研究.1 地质条件鼓2玄区间隧道全长106316m,为双洞单线隧道,线间距1310~1712m,隧洞覆土厚度8~17m.该隧道穿越地层为:粉质粘土、淤泥质粉质粘土、残积粘土、残积质砂粘土和强2微风化安山岩.地下水水位埋深1120m.钻孔揭示区段土层分布如下:①21杂填土:灰褐杂色,湿,松散,厚度013~211m.①22b223素填土:灰褐色,湿,可塑,以粉质粘土为主.厚度015~115m.②21b122粉质粘土:灰黄色,湿,硬2可塑.厚度312~410m.③2121b2粉质粘土:褐黄灰色,湿,可塑.厚度316~613m.③2221b2粉质粘土:灰黄色,可塑.厚度415~516m.③2222b324粉质粘土:灰褐色,很湿,软2流塑.厚度015~118m.③2222b122粉质粘土:灰色,湿,软2流塑.厚度210~410m.⑤2e残积土:棕红色,湿,可塑.厚度115~516 m.K2p21强风化岩:砖红色,原岩为含砾砂岩,经风化呈砂土状.厚度318~613m.2 理论分析与计算211 基本理论 影响隧道地层移动的因素较多,主要有隧道的埋深和直径、地层的物理力学特性、隧道施工参数、支护结构以及周围环境等.一般认为隧道埋深增加,地表沉降槽的宽度系数将增加,地表沉降的最大值减小[223].文献[2]的结论为i=H2πtan45°-φ2(1)式中,i为沉降槽的宽度系数;H为隧道埋深;φ为土体的内摩檫角.文献[3]的结论为i=kD2HDn(2)式中,n为与地层土力学特性及施工因素有关的常数.式(1)、(2)中前者与隧道直径无关,而后者则相关.从具有一定埋深隧道来看,隧道直径对地表沉降情况的影响相对较小.对于因地铁施工引起的地面沉降变形的研究主要包括这两方面:一是由于地铁施工引起的地面沉降变形量和沉降盆地范围的预测[2,4210],主要基于地面最大沉降量计算公式,并假设地面沉降曲线满足高斯分布.计算公式为s=s m ax exp-x22i2(3)式中,sm ax=V12πi为沉降槽的最大沉降量,V1为地层损失量;x.文献[8]考虑土压平衡,提出盾构施工的控制舱压力的经验公式来控制土体扰动变形.文献[11214]采用随机法来预测地铁施工引起的地面沉降.其计算公式为W(x)=κtanβηexp-πtan2βη2(x-ζ)2dζdη(4)二是由于地铁施工对周边环境的引起危害影响研究[15218].为了更准确地预测隧道施工引起的地面沉降,文献[19]通过高斯函数和屈服密度进行弥补预测公式本身的不足(未考虑固结影响).文献[20]考虑土的本构模型用有限元法计算不同隧道开挖的沉降.212 隧道施工固结理论图1为隧道的埋深横断面示意图.以隧道轴心为圆心,径向坐标系下的固结方程为c v1r55r r5u r5r=5u r5t(5)边界条件为u r r=r=0(6)初始条件为u r t=0=r w(r0+H-h0-rcosθ)(7)式中,ur为隧道开挖引起的径向超静孔隙水压力;c v为隧道上部土层的竖向固结系数;r为以隧道轴心为圆心的径向坐标;t为超静孔隙水压力消散时492东南大学学报(自然科学版) 第38卷间;r 0为隧道半径;H 为隧道埋深;h 0为地下水位深度;θ为径向坐标系中圆心角;r w 为地下水容重.图1 隧道的埋深横断面示意图(单位:m )根据定解条件求解方程(5),得到隧道开挖后的周边孔隙水压力为u r (r,t )=∑∞m =12(H +r 0-h 0)cosθ2[J ′0(μm )]2・r 0(r 0+H -h 0)2/cos θμmJ 1μm (H +r 0-h 0)/cosθr 0-r 0μm3cosθJ 1μm (H +r 0-h 0)/cos θr 0μm (H+r 0-h 0)/cosθr02-J 2μm (H +r 0-h 0)/cos θr 0μm (H +r 0-h 0)/cosθr 0e-c v (μm /r 0)2tJ 0μmr 0r(8)式中,J 2(μm ),J 1(μm )和J 0(μm )分别为μm 的二阶、一阶和零阶贝塞尔函数;μm 为J 0(r )的第m 个零点.隧道开挖引起的固结沉降为s (t,θ)=∫(H +r 0-h 0)/cosθ0m v [u rt =0-u r (r,t )]d r(9)式中,m v 为土的体积压缩系数;s (t,θ)为t 时刻与隧道轴心的夹角为θ的地面由于固结造成的沉降.2.3 计算分析采用Plax is 软件对鼓2玄区间隧道在施工中引起的地面沉降进行分析,同时考虑土层损失和土层固结引起的地表沉降(见表1).对相邻的2个隧道断面的地面沉降进行分析,k 11+295顶部覆土厚8185m ,k 11+300顶部覆土厚8171m.图2为有限元网格图,图3为两断面施工前有效应力分布图,图4为两断面施工后有效应力分布图,图5和图6分别为两断面未考虑和考虑固结的计算沉降分布情况.图7为两断面隧道施工引起的地表沉降曲线,计算结果表明,考虑土体固结变形引起的地面沉降计算结果与实测值比较接近和吻合.表1 模拟计算所用土层参数值类型土层厚/m土容重γsat /(kN ・m-3)水平渗透系数K x /(μm ・s -1)垂直渗透系数K z /(μm ・s -1)弹性模量E/M Pa泊松比ν凝聚力C /kPa摩擦角φ/(°)杂填土013201010101015013025101810素填土11019163226.5817013055101215粉质粘土61020143.90.9371201302117817残积土11319141010514013017181417微风化安山岩2010261010102310012118102010图2 有限元网格划分图3 施工前有效应力分布图图4 施工后有效应力分布图592第2期缪林昌,等:城市地铁隧道施工引起的地面沉降图5 仅考虑地层损失引起地面沉降分布图图6 同时考虑地层损失和土层固结地面沉降分布图图7 沿隧道横断面地表沉降曲线3 讨论由于Peck [2]公式没有与具体施工的岩土体性质密切结合起来,也没有考虑地下水水位变化的影响,预测结果与实测结果总有一定的差距.文献[11]提出的随机法来预测地铁施工引起的地面沉降已取得了较好的实用效果,而将固液两相介质有限元分析和随机介质方法相结合,其本质就是要考虑隧道施工过程中岩土体的固结问题.目前国内很多城市隧道施工采用的是矿山法和新奥法,由于施工是分步进行的,在施工过程中必须要采取井点降水或局部降水,开挖施工喷锚加固还有一个时间间隔,因此在研究分析这类工法的隧道施工的环境效应时就需要考虑岩土体的固结引起的地面沉降和变形.虽然可以通过修正系数来调整设计的地面沉降值,但其准确程度就难以得到保证.因此,本文建议同时考虑地层损失和土层固结引起地面沉降对隧道安全设计是必要的.在实际施工过程中,由于地质条件复杂,施工的影响因素也比较多,还需要研究不同土层固结引起的地面沉降占总沉降量的比例,这对隧道施工设计更显重要.在计算时为了简化,计算过程中没有考虑左右两洞不同步的相互影响,实际上在施工时左右开挖不是同步进行的,开挖过程中必然会出现侧向位移,侧向位移大了也会加大垂向位移.这将在以后的计算分析中加以考虑.隧道施工过程和施工后岩土体的固结变形在地表总沉降中所占的比例在各个工程和各地区都将会有所不同,这与施工对土体的扰动程度、施工的工法、降水井点的布置、降水的速度和当地地下水水文地质条件等多种因素有关,同时也与隧道施工过程中周边所遇到的建筑物安全要求等级有关,对于重要的建筑物、历史文物建筑,必须考虑隧道施工引起的土层固结沉降,还要考虑左右洞室的不同步施工引起的侧向位移对地面沉降的影响,这样可能会更安全.4 结语由于地铁施工降水和施工扰动在新的应力状态下产生固结,本文在进行隧道施工的地面沉降分析过程中同时考虑土层的损失和土层固结,并推导出隧道施工过程中土层孔隙水压消散的公式,可计692东南大学学报(自然科学版) 第38卷算孔压消散引起的沉降变形.通过实测沉降数据对比分析,表明考虑土层固结变形后计算结果与实测结果更为吻合,这对设计和施工防范会更安全.参考文献(References)[1]L oganathan N,Poulos H G.A nalytical p rediction fortunneling2induced ground m ovem ents in clays[J].JG eotech G eoenviron Eng,1998,124(9):8462856.[2]Peck R B.D eep excavation and tunneling in soft ground[C]//P roceedings of the7th Interna tiona l C onferenceon Soil M echanics and Founda tion Engineering.M exico,1969:2252290.[3]A ttew ell P B,Far m er I W.G round defor m ations resul2ting from shield tunnelling in L ondon clay[J].C anG eotech J,1974,11:3802395.[4]M air R J,Taylor R N.B ored tunneling in the urban en2vironm ent[C]//P roceedings of14th Interna tiona l Soil M echanics and Founda tion Engineering.B alkem a,Am sterdam,1997,4:235322385.[5]B obet A.A nalytical solutions for shallow tunnels in sat2urated ground[J].J Eng M ech,2001,127(12):125821266.[6]Einstein H H,Schw artz C W.S i m p lified analysis fortunnel supports[J].ASC E J G eotech Eng D iv,1979,104(4):4992518.[7]Park K H.E lastic solution for tunneling2induced groundm ovem ents in clays[J].Interna tiona l J ourna l of G eo2 m echanics,2004,4(4):3102318.[8]孙钧.盾构隧道施工的土体扰动及其环境稳定与控制[C]//第四届海峡两岸都市公共工程学术暨务实研讨会论文集.上海:上海市土木工程学会,1999:3142 333.Sub Jun.Soil disturbance,environm ental stability andcontrolling under shield tunneling[C]//The4th P ro2ceeding of S tra its U rban U tility Engineering and Engi2neering C ases Sy mposium.S hanghai:Society of C ivilEngineering,1999:3142333.(in C hinese)[9]刘建航.上海软土隧道盾构施工技术专家系统综述[J].地下工程与隧道,1995,5(2):126.L iu J ianhang.R eview s of expert system of soft soil tun2nel construction technology in S hanghai[J].U nder2ground Engineering and Tunnels,1995,5(2):126.(inC hinese)[10]侯学渊,廖少明.盾构隧道沉降预估[J].地下工程与隧道,1993(4):24232.H ou X ueyuan,L iao S haom ing.Settlem ent esti m ating ofshield tunnels[J].U nderground Engineering and Tun2nels,1993(4):24232.(in C hinese)[11]刘宝琛,林德璋.浅部隧道开挖引起的地表移动及变形[J].地下工程,1983,3(7):127.L iu B aochen,L in D ezhang.G round surface m ovem entand defor m ation due to shallow tunnel excavation[J].U nderground Space,1983,3(7):127.(in C hinese) [12]刘宝琛,张家生.近地表开挖引起的地表沉降的随机介质方法[J].岩石力学与工程学报,1995,14(4):2892296.L iu B aochen,Zhang J iasheng.S tochastic m ethod forground subsidence due to near surface excavation[J].C hinese J ourna l of Rock M echanics and Engineering,1995,14(4):2892296.(in C hinese)[13]朱忠隆,张庆贺,易宏传.软土隧道纵向地表沉降的随机预测方法[J].岩土力学,2001,22(1):56259.Zhu Zhonglong,Zhang Q inghe,Y i H ongchuan.S to2chastic theory for p redicting longitudinal settlem ent insoft2soil tunnel[J].Rock and Soil M echanics,2001,22(1):56259.(in C hinese)[14]阳军生,刘宝琛.城市隧道施工引起的地表移动与变形[M].北京:中国铁道出版社,2002.[15]B urland J B.A ssessm ent of risk of dam age to build2ings due to tunneling and excavations invited speciallecture[C]//Interna tiona l C onference on Ea rthquakeG eotechnica l Engineering.B alkem a,R otterdam,1995:118921201.[16]H arris D I,M air R J,L ove J P,et al.O bservations ofground and structure m ovem ents for com pensationgrouting during tunnel construction at W aterloo station[J].G eotechnique,1994,44(4):6912713.[17]H arris D I,M air R J,L ove J P,et al.C om pensationgrouting to control tilt of B ig B en C lock Tow er[C]//G eotechnica l Aspects of U nderground C onstruction inSoft G round.B alkem a,R otterdam,1999:2252232. [18]孙钧.地下工程设计理论与实践[M].上海:上海科学技术出版社,1996.[19]C elestino T B,G om es R A M P,B ortloucci A A.Er2rors in ground distortions due to settlem ent trough ad2just m ent[J].Tunnelling and U nderground SpaceTechnology,2000,15(1):972100.[20]Karakus M,Fow ell R J.Effect of different tunnel faceadvance excavation on the settlem ent by FE M[J].Tunnelling and U nderground Space Technology,2003,18(3):5132523.792第2期缪林昌,等:城市地铁隧道施工引起的地面沉降。
土木工程 专业外语词汇大全中英翻译
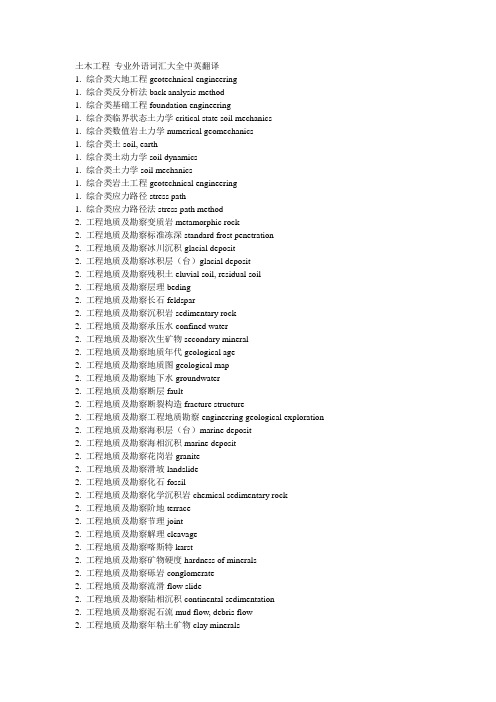
土木工程专业外语词汇大全中英翻译1. 综合类大地工程geotechnical engineering1. 综合类反分析法back analysis method1. 综合类基础工程foundation engineering1. 综合类临界状态土力学critical state soil mechanics1. 综合类数值岩土力学numerical geomechanics1. 综合类土soil, earth1. 综合类土动力学soil dynamics1. 综合类土力学soil mechanics1. 综合类岩土工程geotechnical engineering1. 综合类应力路径stress path1. 综合类应力路径法stress path method2. 工程地质及勘察变质岩metamorphic rock2. 工程地质及勘察标准冻深standard frost penetration2. 工程地质及勘察冰川沉积glacial deposit2. 工程地质及勘察冰积层(台)glacial deposit2. 工程地质及勘察残积土eluvial soil, residual soil2. 工程地质及勘察层理beding2. 工程地质及勘察长石feldspar2. 工程地质及勘察沉积岩sedimentary rock2. 工程地质及勘察承压水confined water2. 工程地质及勘察次生矿物secondary mineral2. 工程地质及勘察地质年代geological age2. 工程地质及勘察地质图geological map2. 工程地质及勘察地下水groundwater2. 工程地质及勘察断层fault2. 工程地质及勘察断裂构造fracture structure2. 工程地质及勘察工程地质勘察engineering geological exploration 2. 工程地质及勘察海积层(台)marine deposit2. 工程地质及勘察海相沉积marine deposit2. 工程地质及勘察花岗岩granite2. 工程地质及勘察滑坡landslide2. 工程地质及勘察化石fossil2. 工程地质及勘察化学沉积岩chemical sedimentary rock2. 工程地质及勘察阶地terrace2. 工程地质及勘察节理joint2. 工程地质及勘察解理cleavage2. 工程地质及勘察喀斯特karst2. 工程地质及勘察矿物硬度hardness of minerals2. 工程地质及勘察砾岩conglomerate2. 工程地质及勘察流滑flow slide2. 工程地质及勘察陆相沉积continental sedimentation2. 工程地质及勘察泥石流mud flow, debris flow2. 工程地质及勘察年粘土矿物clay minerals2. 工程地质及勘察凝灰岩tuff2. 工程地质及勘察牛轭湖ox-bow lake2. 工程地质及勘察浅成岩hypabyssal rock2. 工程地质及勘察潜水ground water2. 工程地质及勘察侵入岩intrusive rock2. 工程地质及勘察取土器geotome2. 工程地质及勘察砂岩sandstone2. 工程地质及勘察砂嘴spit, sand spit2. 工程地质及勘察山岩压力rock pressure2. 工程地质及勘察深成岩plutionic rock2. 工程地质及勘察石灰岩limestone2. 工程地质及勘察石英quartz2. 工程地质及勘察松散堆积物rickle2. 工程地质及勘察围限地下水(台)confined ground water 2. 工程地质及勘察泻湖lagoon2. 工程地质及勘察岩爆rock burst2. 工程地质及勘察岩层产状attitude of rock2. 工程地质及勘察岩浆岩magmatic rock, igneous rock2. 工程地质及勘察岩脉dike, dgke2. 工程地质及勘察岩石风化程度degree of rock weathering 2. 工程地质及勘察岩石构造structure of rock2. 工程地质及勘察岩石结构texture of rock2. 工程地质及勘察岩体rock mass2. 工程地质及勘察页岩shale2. 工程地质及勘察原生矿物primary mineral2. 工程地质及勘察云母mica2. 工程地质及勘察造岩矿物rock-forming mineral2. 工程地质及勘察褶皱fold, folding2. 工程地质及勘察钻孔柱状图bore hole columnar section3. 土的分类饱和土saturated soil3. 土的分类超固结土overconsolidated soil3. 土的分类冲填土dredger fill3. 土的分类充重塑土3. 土的分类冻土frozen soil, tjaele3. 土的分类非饱和土unsaturated soil3. 土的分类分散性土dispersive soil3. 土的分类粉土silt, mo3. 土的分类粉质粘土silty clay3. 土的分类高岭石kaolinite3. 土的分类过压密土(台)overconsolidated soil3. 土的分类红粘土red clay, adamic earth3. 土的分类黄土loess, huangtu(China)3. 土的分类蒙脱石montmorillonite3. 土的分类泥炭peat, bog muck3. 土的分类年粘土clay3. 土的分类年粘性土cohesive soil, clayey soil3. 土的分类膨胀土expansive soil, swelling soil3. 土的分类欠固结粘土underconsolidated soil3. 土的分类区域性土zonal soil3. 土的分类人工填土fill, artificial soil3. 土的分类软粘土soft clay, mildclay, mickle3. 土的分类砂土sand3. 土的分类湿陷性黄土collapsible loess, slumping loess3. 土的分类素填土plain fill3. 土的分类塑性图plasticity chart3. 土的分类碎石土stone, break stone, broken stone, channery, chat, crushed stone, deritus 3. 土的分类未压密土(台)underconsolidated clay3. 土的分类无粘性土cohesionless soil, frictional soil, non-cohesive soil3. 土的分类岩石rock3. 土的分类伊利土illite3. 土的分类有机质土organic soil3. 土的分类淤泥muck, gyttja, mire, slush3. 土的分类淤泥质土mucky soil3. 土的分类原状土undisturbed soil3. 土的分类杂填土miscellaneous fill3. 土的分类正常固结土normally consolidated soil3. 土的分类正常压密土(台)normally consolidated soil3. 土的分类自重湿陷性黄土self weight collapse loess4. 土的物理性质阿太堡界限Atterberg limits4. 土的物理性质饱和度degree of saturation4. 土的物理性质饱和密度saturated density4. 土的物理性质饱和重度saturated unit weight4. 土的物理性质比重specific gravity4. 土的物理性质稠度consistency4. 土的物理性质不均匀系数coefficient of uniformity, uniformity coefficient4. 土的物理性质触变thixotropy4. 土的物理性质单粒结构single-grained structure4. 土的物理性质蜂窝结构honeycomb structure4. 土的物理性质干重度dry unit weight4. 土的物理性质干密度dry density4. 土的物理性质塑性指数plasticity index4. 土的物理性质含水量water content, moisture content4. 土的物理性质活性指数4. 土的物理性质级配gradation, grading4. 土的物理性质结合水bound water, combined water, held water4. 土的物理性质界限含水量Atterberg limits4. 土的物理性质颗粒级配particle size distribution of soils, mechanical composition of soil 4. 土的物理性质可塑性plasticity4. 土的物理性质孔隙比void ratio4. 土的物理性质孔隙率porosity4. 土的物理性质粒度granularity, grainness, grainage4. 土的物理性质粒组fraction, size fraction4. 土的物理性质毛细管水capillary water4. 土的物理性质密度density4. 土的物理性质密实度compactionness4. 土的物理性质年粘性土的灵敏度sensitivity of cohesive soil4. 土的物理性质平均粒径mean diameter, average grain diameter4. 土的物理性质曲率系数coefficient of curvature4. 土的物理性质三相图block diagram, skeletal diagram, three phase diagram4. 土的物理性质三相土tri-phase soil4. 土的物理性质湿陷起始应力initial collapse pressure4. 土的物理性质湿陷系数coefficient of collapsibility4. 土的物理性质缩限shrinkage limit4. 土的物理性质土的构造soil texture4. 土的物理性质土的结构soil structure4. 土的物理性质土粒相对密度specific density of solid particles4. 土的物理性质土中气air in soil4. 土的物理性质土中水water in soil4. 土的物理性质团粒aggregate, cumularpharolith4. 土的物理性质限定粒径constrained diameter4. 土的物理性质相对密度relative density, density index4. 土的物理性质相对压密度relative compaction, compacting factor, percent compaction, coefficient of compaction4. 土的物理性质絮状结构flocculent structure4. 土的物理性质压密系数coefficient of consolidation4. 土的物理性质压缩性compressibility4. 土的物理性质液限liquid limit4. 土的物理性质液性指数liquidity index4. 土的物理性质游离水(台)free water4. 土的物理性质有效粒径effective diameter, effective grain size, effective size4. 土的物理性质有效密度effective density4. 土的物理性质有效重度effective unit weight4. 土的物理性质重力密度unit weight4. 土的物理性质自由水free water, gravitational water, groundwater, phreatic water4. 土的物理性质组构fabric4. 土的物理性质最大干密度maximum dry density4. 土的物理性质最优含水量optimum water content5. 渗透性和渗流达西定律Darcy s law5. 渗透性和渗流管涌piping5. 渗透性和渗流浸润线phreatic line5. 渗透性和渗流临界水力梯度critical hydraulic gradient5. 渗透性和渗流流函数flow function5. 渗透性和渗流流土flowing soil5. 渗透性和渗流流网flow net5. 渗透性和渗流砂沸sand boiling5. 渗透性和渗流渗流seepage5. 渗透性和渗流渗流量seepage discharge5. 渗透性和渗流渗流速度seepage velocity5. 渗透性和渗流渗透力seepage force5. 渗透性和渗流渗透破坏seepage failure5. 渗透性和渗流渗透系数coefficient of permeability5. 渗透性和渗流渗透性permeability5. 渗透性和渗流势函数potential function5. 渗透性和渗流水力梯度hydraulic gradient6. 地基应力和变形变形deformation6. 地基应力和变形变形模量modulus of deformation6. 地基应力和变形泊松比Poisson s ratio6. 地基应力和变形布西涅斯克解Boussinnesq s solution6. 地基应力和变形残余变形residual deformation6. 地基应力和变形残余孔隙水压力residual pore water pressure6. 地基应力和变形超静孔隙水压力excess pore water pressure6. 地基应力和变形沉降settlement6. 地基应力和变形沉降比settlement ratio6. 地基应力和变形次固结沉降secondary consolidation settlement6. 地基应力和变形次固结系数coefficient of secondary consolidation6. 地基应力和变形地基沉降的弹性力学公式elastic formula for settlement calculation 6. 地基应力和变形分层总和法layerwise summation method6. 地基应力和变形负孔隙水压力negative pore water pressure6. 地基应力和变形附加应力superimposed stress6. 地基应力和变形割线模量secant modulus6. 地基应力和变形固结沉降consolidation settlement6. 地基应力和变形规范沉降计算法settlement calculation by specification6. 地基应力和变形回弹变形rebound deformation6. 地基应力和变形回弹模量modulus of resilience6. 地基应力和变形回弹系数coefficient of resilience6. 地基应力和变形回弹指数swelling index6. 地基应力和变形建筑物的地基变形允许值allowable settlement of building6. 地基应力和变形剪胀dilatation6. 地基应力和变形角点法corner-points method6. 地基应力和变形孔隙气压力pore air pressure6. 地基应力和变形孔隙水压力pore water pressure6. 地基应力和变形孔隙压力系数Apore pressure parameter A6. 地基应力和变形孔隙压力系数Bpore pressure parameter B6. 地基应力和变形明德林解Mindlin s solution6. 地基应力和变形纽马克感应图Newmark chart6. 地基应力和变形切线模量tangent modulus6. 地基应力和变形蠕变creep6. 地基应力和变形三向变形条件下的固结沉降three-dimensional consolidation settlement 6. 地基应力和变形瞬时沉降immediate settlement6. 地基应力和变形塑性变形plastic deformation6. 地基应力和变形谈弹性变形elastic deformation6. 地基应力和变形谈弹性模量elastic modulus6. 地基应力和变形谈弹性平衡状态state of elastic equilibrium6. 地基应力和变形体积变形模量volumetric deformation modulus6. 地基应力和变形先期固结压力preconsolidation pressure6. 地基应力和变形压缩层6. 地基应力和变形压缩模量modulus of compressibility6. 地基应力和变形压缩系数coefficient of compressibility6. 地基应力和变形压缩性compressibility6. 地基应力和变形压缩指数compression index6. 地基应力和变形有效应力effective stress6. 地基应力和变形自重应力self-weight stress6. 地基应力和变形总应力total stress approach of shear strength6. 地基应力和变形最终沉降final settlement7. 固结巴隆固结理论Barron s consolidation theory7. 固结比奥固结理论Biot s consolidation theory7. 固结超固结比over-consolidation ratio7. 固结超静孔隙水压力excess pore water pressure7. 固结次固结secondary consolidation7. 固结次压缩(台)secondary consolidatin7. 固结单向度压密(台)one-dimensional consolidation7. 固结多维固结multi-dimensional consolidation7. 固结固结consolidation7. 固结固结度degree of consolidation7. 固结固结理论theory of consolidation7. 固结固结曲线consolidation curve7. 固结固结速率rate of consolidation7. 固结固结系数coefficient of consolidation7. 固结固结压力consolidation pressure7. 固结回弹曲线rebound curve7. 固结井径比drain spacing ratio7. 固结井阻well resistance7. 固结曼代尔-克雷尔效应Mandel-Cryer effect7. 固结潜变(台)creep7. 固结砂井sand drain7. 固结砂井地基平均固结度average degree of consolidation of sand-drained ground7. 固结时间对数拟合法logrithm of time fitting method7. 固结时间因子time factor7. 固结太沙基固结理论Terzaghi s consolidation theory7. 固结太沙基-伦杜列克扩散方程Terzaghi-Rendulic diffusion equation7. 固结先期固结压力preconsolidation pressure7. 固结压密(台)consolidation7. 固结压密度(台)degree of consolidation7. 固结压缩曲线cpmpression curve7. 固结一维固结one dimensional consolidation7. 固结有效应力原理principle of effective stress7. 固结预压密压力(台)preconsolidation pressure7. 固结原始压缩曲线virgin compression curve7. 固结再压缩曲线recompression curve7. 固结主固结primary consolidation7. 固结主压密(台)primary consolidation7. 固结准固结压力pseudo-consolidation pressure7. 固结K0固结consolidation under K0 condition8. 抗剪强度安息角(台)angle of repose8. 抗剪强度不排水抗剪强度undrained shear strength8. 抗剪强度残余内摩擦角residual angle of internal friction8. 抗剪强度残余强度residual strength8. 抗剪强度长期强度long-term strength8. 抗剪强度单轴抗拉强度uniaxial tension test8. 抗剪强度动强度dynamic strength of soils8. 抗剪强度峰值强度peak strength8. 抗剪强度伏斯列夫参数Hvorslev parameter8. 抗剪强度剪切应变速率shear strain rate8. 抗剪强度抗剪强度shear strength8. 抗剪强度抗剪强度参数shear strength parameter8. 抗剪强度抗剪强度有效应力法effective stress approach of shear strength 8. 抗剪强度抗剪强度总应力法total stress approach of shear strength8. 抗剪强度库仑方程Coulomb s equation8. 抗剪强度摩尔包线Mohr s envelope8. 抗剪强度摩尔-库仑理论Mohr-Coulomb theory8. 抗剪强度内摩擦角angle of internal friction8. 抗剪强度年粘聚力cohesion8. 抗剪强度破裂角angle of rupture8. 抗剪强度破坏准则failure criterion8. 抗剪强度十字板抗剪强度vane strength8. 抗剪强度无侧限抗压强度unconfined compression strength8. 抗剪强度有效内摩擦角effective angle of internal friction8. 抗剪强度有效粘聚力effective cohesion intercept8. 抗剪强度有效应力破坏包线effective stress failure envelope8. 抗剪强度有效应力强度参数effective stress strength parameter8. 抗剪强度有效应力原理principle of effective stress8. 抗剪强度真内摩擦角true angle internal friction8. 抗剪强度真粘聚力true cohesion8. 抗剪强度总应力破坏包线total stress failure envelope8. 抗剪强度总应力强度参数total stress strength parameter9. 本构模型本构模型constitutive model9. 本构模型边界面模型boundary surface model9. 本构模型层向各向同性体模型cross anisotropic model9. 本构模型超弹性模型hyperelastic model9. 本构模型德鲁克-普拉格准则Drucker-Prager criterion9. 本构模型邓肯-张模型Duncan-Chang model9. 本构模型动剪切强度9. 本构模型非线性弹性模量nonlinear elastic model9. 本构模型盖帽模型cap model9. 本构模型刚塑性模型rigid plastic model9. 本构模型割线模量secant modulus9. 本构模型广义冯·米赛斯屈服准则extended von Mises yield criterion 9. 本构模型广义特雷斯卡屈服准则extended tresca yield criterion9. 本构模型加工软化work softening9. 本构模型加工硬化work hardening9. 本构模型加工硬化定律strain harding law9. 本构模型剑桥模型Cambridge model9. 本构模型柯西弹性模型Cauchy elastic model9. 本构模型拉特-邓肯模型Lade-Duncan model9. 本构模型拉特屈服准则Lade yield criterion9. 本构模型理想弹塑性模型ideal elastoplastic model9. 本构模型临界状态弹塑性模型critical state elastoplastic model9. 本构模型流变学模型rheological model9. 本构模型流动规则flow rule9. 本构模型摩尔-库仑屈服准则Mohr-Coulomb yield criterion9. 本构模型内蕴时间塑性模型endochronic plastic model9. 本构模型内蕴时间塑性理论endochronic theory9. 本构模型年粘弹性模型viscoelastic model9. 本构模型切线模量tangent modulus9. 本构模型清华弹塑性模型Tsinghua elastoplastic model9. 本构模型屈服面yield surface9. 本构模型沈珠江三重屈服面模型Shen Zhujiang three yield surface method 9. 本构模型双参数地基模型9. 本构模型双剪应力屈服模型twin shear stress yield criterion9. 本构模型双曲线模型hyperbolic model9. 本构模型松岗元-中井屈服准则Matsuoka-Nakai yield criterion9. 本构模型塑性形变理论9. 本构模型谈弹塑性模量矩阵elastoplastic modulus matrix9. 本构模型谈弹塑性模型elastoplastic modulus9. 本构模型谈弹塑性增量理论incremental elastoplastic theory9. 本构模型谈弹性半空间地基模型elastic half-space foundation model9. 本构模型谈弹性变形elastic deformation9. 本构模型谈弹性模量elastic modulus9. 本构模型谈弹性模型elastic model9. 本构模型魏汝龙-Khosla-Wu模型Wei Rulong-Khosla-Wu model9. 本构模型文克尔地基模型Winkler foundation model9. 本构模型修正剑桥模型modified cambridge model9. 本构模型准弹性模型hypoelastic model10. 地基承载力冲剪破坏punching shear failure10. 地基承载力次层(台)substratum10. 地基承载力地基subgrade, ground, foundation soil10. 地基承载力地基承载力bearing capacity of foundation soil10. 地基承载力地基极限承载力ultimate bearing capacity of foundation soil10. 地基承载力地基允许承载力allowable bearing capacity of foundation soil10. 地基承载力地基稳定性stability of foundation soil10. 地基承载力汉森地基承载力公式Hansen s ultimate bearing capacity formula10. 地基承载力极限平衡状态state of limit equilibrium10. 地基承载力加州承载比(美国)California Bearing Ratio10. 地基承载力局部剪切破坏local shear failure10. 地基承载力临塑荷载critical edge pressure10. 地基承载力梅耶霍夫极限承载力公式Meyerhof s ultimate bearing capacity formula 10. 地基承载力普朗特承载力理论Prandel bearing capacity theory10. 地基承载力斯肯普顿极限承载力公式Skempton s ultimate bearing capacity formula 10. 地基承载力太沙基承载力理论Terzaghi bearing capacity theory10. 地基承载力魏锡克极限承载力公式V esic s ultimate bearing capacity formula10. 地基承载力整体剪切破坏general shear failure11. 土压力被动土压力passive earth pressure11. 土压力被动土压力系数coefficient of passive earth pressure11. 土压力极限平衡状态state of limit equilibrium11. 土压力静止土压力earth pressue at rest11. 土压力静止土压力系数coefficient of earth pressur at rest11. 土压力库仑土压力理论Coulomb s earth pressure theory11. 土压力库尔曼图解法Culmannn construction11. 土压力朗肯土压力理论Rankine s earth pressure theory11. 土压力朗肯状态Rankine state11. 土压力谈弹性平衡状态state of elastic equilibrium11. 土压力土压力earth pressure11. 土压力主动土压力active earth pressure11. 土压力主动土压力系数coefficient of active earth pressure12. 土坡稳定分析安息角(台)angle of repose12. 土坡稳定分析毕肖普法Bishop method12. 土坡稳定分析边坡稳定安全系数safety factor of slope12. 土坡稳定分析不平衡推理传递法unbalanced thrust transmission method12. 土坡稳定分析费伦纽斯条分法Fellenius method of slices12. 土坡稳定分析库尔曼法Culmann method12. 土坡稳定分析摩擦圆法friction circle method12. 土坡稳定分析摩根斯坦-普拉斯法Morgenstern-Price method12. 土坡稳定分析铅直边坡的临界高度critical height of vertical slope12. 土坡稳定分析瑞典圆弧滑动法Swedish circle method12. 土坡稳定分析斯宾赛法Spencer method12. 土坡稳定分析泰勒法Taylor method12. 土坡稳定分析条分法slice method12. 土坡稳定分析土坡slope12. 土坡稳定分析土坡稳定分析slope stability analysis12. 土坡稳定分析土坡稳定极限分析法limit analysis method of slope stability 12. 土坡稳定分析土坡稳定极限平衡法limit equilibrium method of slope stability 12. 土坡稳定分析休止角angle of repose12. 土坡稳定分析扬布普遍条分法Janbu general slice method12. 土坡稳定分析圆弧分析法circular arc analysis13. 土的动力性质比阻尼容量specific gravity capacity13. 土的动力性质波的弥散特性dispersion of waves13. 土的动力性质波速法wave velocity method13. 土的动力性质材料阻尼material damping13. 土的动力性质初始液化initial liquefaction13. 土的动力性质地基固有周期natural period of soil site13. 土的动力性质动剪切模量dynamic shear modulus of soils13. 土的动力性质动力布西涅斯克解dynamic solution of Boussinesq13. 土的动力性质动力放大因素dynamic magnification factor13. 土的动力性质动力性质dynamic properties of soils13. 土的动力性质动强度dynamic strength of soils13. 土的动力性质骨架波akeleton waves in soils13. 土的动力性质几何阻尼geometric damping13. 土的动力性质抗液化强度liquefaction stress13. 土的动力性质孔隙流体波fluid wave in soil13. 土的动力性质损耗角loss angle13. 土的动力性质往返活动性reciprocating activity13. 土的动力性质无量纲频率dimensionless frequency13. 土的动力性质液化liquefaction13. 土的动力性质液化势评价evaluation of liquefaction potential13. 土的动力性质液化应力比stress ratio of liquefaction13. 土的动力性质应力波stress waves in soils13. 土的动力性质振陷dynamic settlement13. 土的动力性质阻尼damping of soil13. 土的动力性质阻尼比damping ratio14. 挡土墙挡土墙retaining wall14. 挡土墙挡土墙排水设施14. 挡土墙挡土墙稳定性stability of retaining wall14. 挡土墙垛式挡土墙14. 挡土墙扶垛式挡土墙counterfort retaining wall14. 挡土墙后垛墙(台)counterfort retaining wall14. 挡土墙基础墙foundation wall14. 挡土墙加筋土挡墙reinforced earth bulkhead14. 挡土墙锚定板挡土墙anchored plate retaining wall14. 挡土墙锚定式板桩墙anchored sheet pile wall14. 挡土墙锚杆式挡土墙anchor rod retaining wall14. 挡土墙悬壁式板桩墙cantilever sheet pile wall14. 挡土墙悬壁式挡土墙cantilever sheet pile wall14. 挡土墙重力式挡土墙gravity retaining wall15. 板桩结构物板桩sheet pile15. 板桩结构物板桩结构sheet pile structure15. 板桩结构物钢板桩steel sheet pile15. 板桩结构物钢筋混凝土板桩reinforced concrete sheet pile15. 板桩结构物钢桩steel pile15. 板桩结构物灌注桩cast-in-place pile15. 板桩结构物拉杆tie rod15. 板桩结构物锚定式板桩墙anchored sheet pile wall15. 板桩结构物锚固技术anchoring15. 板桩结构物锚座Anchorage15. 板桩结构物木板桩wooden sheet pile15. 板桩结构物木桩timber piles15. 板桩结构物悬壁式板桩墙cantilever sheet pile wall16. 基坑开挖与降水板桩围护sheet pile-braced cuts16. 基坑开挖与降水电渗法electro-osmotic drainage16. 基坑开挖与降水管涌piping16. 基坑开挖与降水基底隆起heave of base16. 基坑开挖与降水基坑降水dewatering16. 基坑开挖与降水基坑失稳instability (failure) of foundation pit16. 基坑开挖与降水基坑围护bracing of foundation pit16. 基坑开挖与降水减压井relief well16. 基坑开挖与降水降低地下水位法dewatering method16. 基坑开挖与降水井点系统well point system16. 基坑开挖与降水喷射井点eductor well point16. 基坑开挖与降水铅直边坡的临界高度critical height of vertical slope 16. 基坑开挖与降水砂沸sand boiling16. 基坑开挖与降水深井点deep well point16. 基坑开挖与降水真空井点vacuum well point16. 基坑开挖与降水支撑围护braced cuts17. 浅基础杯形基础17. 浅基础补偿性基础compensated foundation17. 浅基础持力层bearing stratum17. 浅基础次层(台)substratum17. 浅基础单独基础individual footing17. 浅基础倒梁法inverted beam method17. 浅基础刚性角pressure distribution angle of masonary foundation 17. 浅基础刚性基础rigid foundation17. 浅基础高杯口基础17. 浅基础基础埋置深度embeded depth of foundation17. 浅基础基床系数coefficient of subgrade reaction17. 浅基础基底附加应力net foundation pressure17. 浅基础交叉条形基础cross strip footing17. 浅基础接触压力contact pressure17. 浅基础静定分析法(浅基础)static analysis (shallow foundation)17. 浅基础壳体基础shell foundation17. 浅基础扩展基础spread footing17. 浅基础片筏基础mat foundation17. 浅基础浅基础shallow foundation17. 浅基础墙下条形基础17. 浅基础热摩奇金法Zemochkin s method17. 浅基础柔性基础flexible foundation17. 浅基础上部结构-基础-土共同作用分析structure- foundation-soil interactionanalysis 17. 浅基础谈弹性地基梁(板)分析analysis of beams and slabs on elastic foundation 17. 浅基础条形基础strip footing17. 浅基础下卧层substratum17. 浅基础箱形基础box foundation17. 浅基础柱下条形基础18. 深基础贝诺托灌注桩Benoto cast-in-place pile18. 深基础波动方程分析Wave equation analysis18. 深基础场铸桩(台)cast-in-place pile18. 深基础沉管灌注桩diving casting cast-in-place pile18. 深基础沉井基础open-end caisson foundation18. 深基础沉箱基础box caisson foundation18. 深基础成孔灌注同步桩synchronous pile18. 深基础承台pile caps18. 深基础充盈系数fullness coefficient18. 深基础单桩承载力bearing capacity of single pile18. 深基础单桩横向极限承载力ultimate lateral resistance of single pile18. 深基础单桩竖向抗拔极限承载力vertical ultimate uplift resistance of single pile18. 深基础单桩竖向抗压容许承载力vertical ultimate carrying capacity of single pile18. 深基础单桩竖向抗压极限承载力vertical allowable load capacity of single pile18. 深基础低桩承台low pile cap18. 深基础地下连续墙diaphgram wall18. 深基础点承桩(台)end-bearing pile18. 深基础动力打桩公式dynamic pile driving formula18. 深基础端承桩end-bearing pile18. 深基础法兰基灌注桩Franki pile18. 深基础负摩擦力negative skin friction of pile18. 深基础钢筋混凝土预制桩precast reinforced concrete piles18. 深基础钢桩steel pile18. 深基础高桩承台high-rise pile cap18. 深基础灌注桩cast-in-place pile18. 深基础横向载荷桩laterally loaded vertical piles18. 深基础护壁泥浆slurry coat method18. 深基础回转钻孔灌注桩rotatory boring cast-in-place pile18. 深基础机挖异形灌注桩18. 深基础静力压桩silent piling18. 深基础抗拔桩uplift pile18. 深基础抗滑桩anti-slide pile18. 深基础摩擦桩friction pile18. 深基础木桩timber piles18. 深基础嵌岩灌注桩piles set into rock18. 深基础群桩pile groups18. 深基础群桩效率系数efficiency factor of pile groups18. 深基础群桩效应efficiency of pile groups18. 深基础群桩竖向极限承载力vertical ultimate load capacity of pile groups 18. 深基础深基础deep foundation18. 深基础竖直群桩横向极限承载力18. 深基础无桩靴夯扩灌注桩rammed bulb ile18. 深基础旋转挤压灌注桩18. 深基础桩piles18. 深基础桩基动测技术dynamic pile test18. 深基础钻孔墩基础drilled-pier foundation18. 深基础钻孔扩底灌注桩under-reamed bored pile18. 深基础钻孔压注桩starsol enbesol pile18. 深基础最后贯入度final set19. 地基处理表层压密法surface compaction19. 地基处理超载预压surcharge preloading19. 地基处理袋装砂井sand wick19. 地基处理地工织物geofabric, geotextile19. 地基处理地基处理ground treatment, foundation treatment19. 地基处理电动化学灌浆electrochemical grouting19. 地基处理电渗法electro-osmotic drainage19. 地基处理顶升纠偏法19. 地基处理定喷directional jet grouting19. 地基处理冻土地基处理frozen foundation improvement19. 地基处理短桩处理treatment with short pile19. 地基处理堆载预压法preloading19. 地基处理粉体喷射深层搅拌法powder deep mixing method19. 地基处理复合地基composite foundation19. 地基处理干振成孔灌注桩vibratory bored pile19. 地基处理高压喷射注浆法jet grounting19. 地基处理灌浆材料injection material19. 地基处理灌浆法grouting19. 地基处理硅化法silicification19. 地基处理夯实桩compacting pile19. 地基处理化学灌浆chemical grouting19. 地基处理换填法cushion19. 地基处理灰土桩lime soil pile19. 地基处理基础加压纠偏法19. 地基处理挤密灌浆compaction grouting19. 地基处理挤密桩compaction pile, compacted column19. 地基处理挤淤法displacement method19. 地基处理加筋法reinforcement method19. 地基处理加筋土reinforced earth19. 地基处理碱液法soda solution grouting19. 地基处理浆液深层搅拌法grout deep mixing method19. 地基处理降低地下水位法dewatering method19. 地基处理纠偏技术19. 地基处理坑式托换pit underpinning19. 地基处理冷热处理法freezing and heating19. 地基处理锚固技术anchoring19. 地基处理锚杆静压桩托换anchor pile underpinning19. 地基处理排水固结法consolidation19. 地基处理膨胀土地基处理expansive foundation treatment19. 地基处理劈裂灌浆fracture grouting19. 地基处理浅层处理shallow treatment19. 地基处理强夯法dynamic compaction19. 地基处理人工地基artificial foundation19. 地基处理容许灌浆压力allowable grouting pressure19. 地基处理褥垫pillow19. 地基处理软土地基soft clay ground19. 地基处理砂井sand drain19. 地基处理砂井地基平均固结度average degree of consolidation of sand-drained ground 19. 地基处理砂桩sand column19. 地基处理山区地基处理foundation treatment in mountain area19. 地基处理深层搅拌法deep mixing method19. 地基处理渗入性灌浆seep-in grouting19. 地基处理湿陷性黄土地基处理collapsible loess treatment19. 地基处理石灰系深层搅拌法lime deep mixing method19. 地基处理石灰桩lime column, limepile19. 地基处理树根桩root pile19. 地基处理水泥土水泥掺合比cement mixing ratio19. 地基处理水泥系深层搅拌法cement deep mixing method19. 地基处理水平旋喷horizontal jet grouting19. 地基处理塑料排水带plastic drain19. 地基处理碎石桩gravel pile, stone pillar19. 地基处理掏土纠偏法19. 地基处理天然地基natural foundation19. 地基处理土工聚合物Geopolymer19. 地基处理土工织物geofabric, geotextile19. 地基处理土桩earth pile19. 地基处理托换技术underpinning technique19. 地基处理外掺剂additive19. 地基处理旋喷jet grouting19. 地基处理药液灌浆chemical grouting19. 地基处理预浸水法presoaking19. 地基处理预压法preloading19. 地基处理真空预压vacuum preloading19. 地基处理振冲法vibroflotation method19. 地基处理振冲密实法vibro-compaction19. 地基处理振冲碎石桩vibro replacement stone column19. 地基处理振冲置换法vibro-replacement19. 地基处理振密、挤密法vibro-densification, compacting19. 地基处理置换率(复合地基)replacement ratio19. 地基处理重锤夯实法tamping19. 地基处理桩式托换pile underpinning19. 地基处理桩土应力比stress ratio20. 动力机器基础比阻尼容量specific gravity capacity20. 动力机器基础等效集总参数法constant strain rate consolidation test20. 动力机器基础地基固有周期natural period of soil site20. 动力机器基础动基床反力法dynamic subgrade reaction method20. 动力机器基础动力放大因素dynamic magnification factor20. 动力机器基础隔振isolation20. 动力机器基础基础振动foundation vibration20. 动力机器基础基础振动半空间理论elastic half-space theory of foundation vibr ation20. 动力机器基础基础振动容许振幅allowable amplitude of foundation vibration 20. 动力机器基础基础自振频率natural frequency of foundation20. 动力机器基础集总参数法lumped parameter method20. 动力机器基础吸收系数absorption coefficient20. 动力机器基础质量-弹簧-阻尼器系统mass-spring-dushpot system21. 地基基础抗震地基固有周期natural period of soil site21. 地基基础抗震地震earthquake, seism, temblor21. 地基基础抗震地震持续时间duration of earthquake21. 地基基础抗震地震等效均匀剪应力equivalent even shear stress of earthquake 21. 地基基础抗震地震反应谱earthquake response spectrum21. 地基基础抗震地震烈度earthquake intensity21. 地基基础抗震地震震级earthquake magnitude21. 地基基础抗震地震卓越周期seismic predominant period21. 地基基础抗震地震最大加速度maximum acceleration of earthquake21. 地基基础抗震动力放大因数dynamic magnification factor21. 地基基础抗震对数递减率logrithmic decrement21. 地基基础抗震刚性系数coefficient of rigidity21. 地基基础抗震吸收系数absorption coefficient22. 室内土工试验比重试验specific gravity test22. 室内土工试验变水头渗透试验falling head permeability test22. 室内土工试验不固结不排水试验unconsolidated-undrained triaxial test22. 室内土工试验常规固结试验routine consolidation test22. 室内土工试验常水头渗透试验constant head permeability test22. 室内土工试验单剪仪simple shear apparatus22. 室内土工试验单轴拉伸试验uniaxial tensile test22. 室内土工试验等速加荷固结试验constant loading rate consolidatin test22. 室内土工试验等梯度固结试验constant gradient consolidation test22. 室内土工试验等应变速率固结试验equivalent lumped parameter method22. 室内土工试验反复直剪强度试验repeated direct shear test22. 室内土工试验反压饱和法back pressure saturation method22. 室内土工试验高压固结试验high pressure consolidation test22. 室内土工试验各向不等压固结不排水试验consoidated anisotropically undrained test 22. 室内土工试验各向不等压固结排水试验consolidated anisotropically drained test 22. 室内土工试验共振柱试验resonant column test22. 室内土工试验固结不排水试验consolidated undrained triaxial test22. 室内土工试验固结快剪试验consolidated quick direct shear test22. 室内土工试验固结排水试验consolidated drained triaxial test22. 室内土工试验固结试验consolidation test22. 室内土工试验含水量试验water content test22. 室内土工试验环剪试验ring shear test22. 室内土工试验黄土湿陷试验loess collapsibility test22. 室内土工试验击实试验22. 室内土工试验界限含水量试验Atterberg limits test22. 室内土工试验卡萨格兰德法Casagrande s method22. 室内土工试验颗粒分析试验grain size analysis test22. 室内土工试验孔隙水压力消散试验pore pressure dissipation test22. 室内土工试验快剪试验quick direct shear test22. 室内土工试验快速固结试验fast consolidation test22. 室内土工试验离心模型试验centrifugal model test22. 室内土工试验连续加荷固结试验continual loading test22. 室内土工试验慢剪试验consolidated drained direct shear test22. 室内土工试验毛细管上升高度试验capillary rise test22. 室内土工试验密度试验density test22. 室内土工试验扭剪仪torsion shear apparatus22. 室内土工试验膨胀率试验swelling rate test22. 室内土工试验平面应变仪plane strain apparatus22. 室内土工试验三轴伸长试验triaxial extension test22. 室内土工试验三轴压缩试验triaxial compression test22. 室内土工试验砂的相对密实度试验sand relative density test22. 室内土工试验筛分析sieve analysis。
地表沉降研究方法英语作文

地表沉降研究方法英语作文Title: Research Methods for Land Subsidence。
Land subsidence, the gradual sinking of the Earth's surface, is a complex phenomenon with multifaceted causes and impacts. Understanding and mitigating land subsidence require comprehensive research methodologies. In this essay, we delve into various research methods employed to study land subsidence.1. Geodetic Surveys: Geodetic surveys involve precise measurements of the Earth's surface using techniques suchas GPS (Global Positioning System), GNSS (Global Navigation Satellite System), and leveling. These surveys provide valuable data on ground deformation over time, aiding inthe identification and monitoring of subsidence-prone areas.2. Interferometric Synthetic Aperture Radar (InSAR): InSAR is a remote sensing technique that utilizessatellite-based radar to detect ground movements withmillimeter-scale accuracy. By analyzing interferograms generated from multiple radar images, researchers can map land subsidence over large areas and monitor its spatial and temporal evolution.3. Groundwater Monitoring: Land subsidence oftenresults from excessive groundwater extraction, causing aquifer compaction and subsequent surface sinking. Groundwater monitoring involves measuring water levels in wells and aquifers to assess groundwater depletion rates and its correlation with subsidence.4. Geotechnical Investigations: Geotechnical investigations focus on understanding the subsurface soil properties and geological conditions that contribute to land subsidence. Techniques such as borehole drilling, soil sampling, and geophysical surveys help characterize the subsurface and identify potential triggers of subsidence, such as clay layer compression or underground mining activities.5. Numerical Modeling: Numerical modeling plays acrucial role in simulating and predicting land subsidence phenomena. Finite element method (FEM) and finitedifference method (FDM) are commonly used numerical techniques to simulate groundwater flow, soil deformation, and surface subsidence processes. These models integrate geospatial data, hydrogeological parameters, and mechanical properties to simulate the complex interactions driving land subsidence.6. Remote Sensing and Geographic Information Systems (GIS): Remote sensing data, including optical imagery, thermal infrared, and LiDAR (Light Detection and Ranging), provide valuable information for land subsidence analysis. GIS platforms facilitate spatial data management, visualization, and spatial analysis, enabling researchers to integrate multi-source data for comprehensive subsidence assessment.7. Satellite Altimetry: Satellite altimetry measures variations in sea surface height, which indirectly reflect changes in land elevation due to subsidence. By analyzing long-term satellite altimetry data, researchers canestimate regional land subsidence rates in coastal areas and low-lying regions affected by sea-level rise and groundwater extraction.8. Collaborative Monitoring Networks: Collaborative monitoring networks involve partnerships between government agencies, research institutions, and local communities to establish comprehensive subsidence monitoring programs. These networks leverage resources, expertise, and data-sharing mechanisms to enhance subsidence research, raise public awareness, and support informed decision-making for sustainable land use planning.In conclusion, the study of land subsidence requires a multidisciplinary approach encompassing geodesy, remote sensing, hydrogeology, geotechnical engineering, and numerical modeling. By employing an array of research methods, scientists can gain insights into the causes, mechanisms, and impacts of land subsidence, ultimately contributing to effective mitigation strategies and sustainable land management practices.。
外文资料翻译---柔弱岩石上短距离隧道的动态施工力学的研究

毕业设计外文资料翻译题目柔弱岩石上短距离隧道的动态施工力学的研究学院土木建筑学院专业土木工程班级土木学生二〇一一年三月四日Modern Applied Science V ol. 4, No. 6; June 2010 柔弱岩石上短距离隧道的动态施工力学的研究吴恒斌(通讯作者)重庆长江三峡大学土木工程系中国重庆万州市二段沙龙路780号电子邮件:hbw8456@贺云翔重庆长江三峡大学土木工程系中国重庆万州市二段沙龙路780号郭良松聊城建宇工程有限公司中国聊城252000摘要基于建设理论的新奥地利方法((NAM)),依赖在柔弱岩石的短距离隧道工程,通过构建数学模型并进行了三维弹塑性有限元法的建构过程中,双边墙的施工方法。
分析隧道周围一些测量点位移的变化和隧道开挖和洞室群围岩的稳定性,通过分析地表塌陷、承担的力量支护结构与塑性区。
结果表明,上述构造法是合理的在以后的隧道开挖,地表沉陷,隧道变形与早期隧道开挖的影响比较明显。
关键词:柔弱的岩石、小距离隧道、动态建筑机械、数值模拟1介绍过程的开挖与支护隧道是一项复杂的机械加工过程,施工过程之间的差别,开挖顺序,支持的时间大为影响工程结构系统(SHE et al., 2006).的力学效应由于周围岩石条件的复杂性普通的类似项目在柔弱岩石特别是小距离隧道工程的复杂连接中是不够的,因此,根据在施工过程中各负荷情况,在不同的围岩中有必要进行机械模拟和分析在柔弱岩石隧道衬砌方面的研究,SUN et al. (1994)考虑了时空效应隧道挖掘表面建立三维数模型。
CHENG et al. (1997) 分析了力学机制和FLAC隧道衬里复杂的承载能力,得到一些有用的结论。
JIN et al. (1996) 应用非线性粘弹性理论进行了三维有限元模拟圆隧道开挖过程。
Karakus(2007)阐述了由平面应变分析造成的三个尺寸挖掘影响。
因为时空效应还不能全部体现,许多研究人员进行了三维弹塑性有限元法和隧道开挖的弹塑性分析(AN, 1994, XIAO, 2000 & ZHU et al. 1996)。
土木工程 建筑 外文翻译 外文文献 英文文献 地铁地表沉降

外文原文Surface settlement predictions for Istanbul Metro tunnelsexcavated by EPB-TBMS. G. Ercelebi • H. Copur • I. OcakAbstract In this study, short-term surface settlements are predicted for twin tunnels, which are to be excavated in the chainage of 0 ? 850 to 0 ? 900 m between the Esenler and Kirazlı stations of the Istanbul Metro line, which is 4 km in length. The total length of the excavation line is 21.2 km between Esenler and Basaksehir. Tunnels are excavated by employing two earth pressure balance (EPB) tunnel boring machines (TBMs) that have twin tubes of 6.5 m diameter and with 14 m distance from center to center. The TBM in the right tube follows about 100 m behind the other tube. Segmental lining of 1.4 m length is currently employed as the final support. Settlement predictions are performed with finite element method by using Plaxis finite element program. Excavation, ground support and face support steps in FEM analyses are simulated as applied in the field. Predictions are performed for a typicalgeological zone, which is considered as critical in terms of surface settlement. Geology in the study area is composed of fill, very stiff clay, dense sand, very dense sand and hard clay, respectively, starting from the surface. In addition to finite element modeling, the surface settlements are also predicted by using semi-theoretical (semi-empirical) and analytical methods. The results indicate that the FE model predicts well the short-term surface settlements for a given volume loss value. The results of semi-theoretical and analytical methods are found to be in good agreement with the FE model. The results of predictions are compared and verified by field measurements. It is suggested that grouting of the excavation void should be performed as fast as possible after excavation of a section as a precaution against surface settlements during excavation. Face pressure of the TBMs should be closely monitored and adjusted for different zones.Keywords Surface settlement prediction _ Finite element method _ Analytical method _ Semi-theoretical method _ EPB-TBM tunneling _Istanbul MetroIntroductionIncreasing demand on infrastructures increases attention to shallow soft ground tunneling methods in urbanized areas. Many surface and sub-surface structures make underground construction works very delicate due to the influence of ground deformation, which should be definitely limited/controlled to acceptable levels. Independent of theexcavation method, the short- and long-term surface and sub-surface ground deformations should be predicted and remedial precautions against any damage to existing structures planned prior to construction. Tunneling cost substantially increases due to damages to structures resulting from surface settlements, which are above tolerable limits (Bilgin et al. 2021).Basic parameters affecting the ground deformations are ground conditions, technical/environmental parameters and tunneling or construction methods (O’Reilly and New 1982; Arioglu 1992; Karakus and Fowell 2003; Tan and Ranj it 2003; Minguez et al. 2005; Ellis 2005; Suwansawat and Einstein 2006). A thorough study of the ground by site investigations should be performed to find out the physical and mechanical properties of the ground and existence ofunderground water, as well as deformation characteristics, especially the stiffness. Technical parameters include tunnel depth and geometry, tunnel diameter–line–grade, single or double track lines and neighboring structures. The construction method, which should lead to a safe and economic project, is selected based on site characteristics and technical project constraints and should be planned so that the ground movements are limited to an acceptablelevel. Excavation method, face support pressure, advance (excavation) rate, stiffness of support system, excavation sequence and ground treatment/improvement have dramatic effects on the ground deformations occurring due to tunneling operations. The primary reason for ground movements above the tunnel, also known as surface settlements, is convergence of the ground into the tunnel after excavation, which changes the in situ stress state of the ground and results in stress relief. Convergence of the ground is also known as ground loss or volume loss. The volume of the settlement on the surface is usually assumed to be equal to the ground (volume) loss inside the tunnel (O’Reilly and New 1982).Ground loss can be classified as radial loss around the tunnel periphery and axial (face) loss at the excavation face (Attewell et al. 1986; Schmidt 1974). The exact ratio of radial and axial volume losses is not fully demonstrated or generalized in any study. However, it is possible to diminish or minimize the face loss in full-face mechanized excavations by applying a face pressure as a slurry of bentonite–water mixture or foam-processed muck. The ground loss is usually more in granular soils than in cohesive soils for similar construction conditions. The width of the settlement trough on both sides of the tunnel axis is wider in the case of cohesive soils, which means lower maximum settlement for the same amount of ground loss.Time dependency of ground behavior and existence of underground water distinguish short- and long-term settlements (Attewell et al. 1986). Short-term settlements occur during or after a few days (mostly a few weeks) of excavation, assuming that undrained soil conditions are dominant. Long-term settlements are mostly due to creep, stress redistribution and consolidation of soil after drainageof the underground water and elimination of pore water pressure inside the soil, and it may take a few months to a few years to reach a stabilized level. In dry soil conditions, the long-term settlements may be considered as very limited.There are mainly three settlement prediction approaches for mechanized tunnelexcavations: (1) numerical analysis such as finite element method, (2) analytical method and (3) semi-theoretical (semi-empirical) method. Among them, the numerical approaches are the most reliable ones. However, the results of all methods should be used carefully by an experienced field engineer in designing the stage of an excavation project.In this study, all three prediction methods are employed for a critical zone to predict the short-term maximum surface settlements above the twin tunnels of the chainage between 0 ? 850 and 0 ? 900 m between Esenler and Kirazlıstations of Istanbul Metro line, which is 4 km in length. Plaxis finite element modeling program is used fornumerical modeling; the method suggested by Loganathan and Poulos (1998) is used for the analytical solution. A few different semi-theoretical models are also used for predictions. The results are compared and validated by field measurements. Description of the project, site and construction methodThe first construction phase of Istanbul Metro line was started in 1992 and opened to public in 2000. This line is being extended gradually, as well as new lines are being constructed in other locations. One of these metro lines is the twin line between Esenler and Basaksehir, which is 21.2 km. The excavation of this section has been started in May 2006. Currently, around 1,400 m of excavationhas already been completed. The region is highly populated including several story buildings, industrial zones and heavy traffic. Alignment and stations of the metro line between Esenler and Basaksehir is presented in Fig. 1.Totally four earth pressure balance (EPB) tunnel boring machines (TBM) are used for excavation of the tunnels. The metro lines in the study area are excavated by a Herrenknecht EPB-TBM in the right tube and a Lovat EPB-TBM in the left tube. Right tube excavationfollows around 100 m behind the left tube. Some of the technical features of the machines are summarized in Table 1.Excavated material is removed by auger (screw conveyor) through the machine to a belt conveyor and than loaded to rail cars for transporting to the portal. Since the excavated ground bears water and includes stability problems, the excavation chamber is pressurized by 300 kPa and conditioned by applying water, foam, bentonite and polymers through the injection ports. Chamber pressure is continuously monitored by pressure sensors inside thechamber and auger. Installation of a segment ring with 1.4-m length (inner diameter of 5.7 m and outer diameter of 6.3 m) and 30-cm thickness is realized by a wing-type vacuum erector. The ring is configured as five segments plus a key segment. After installation of the ring, the excavation restarts and the void between the segment outer perimeter and excavated tunnel perimeter is grouted by300 kPa of pressure through the grout cannels in the trailing shield. This method of construction has beenproven to minimize the surface settlements.The study area includes the twin tunnels of the chainage between 0 + 850 and 0 + 900 m, between Esenler and Kirazlı stations. Gungoren Formation of the Miosen age is found in the study area. Laboratory and in situ tests are applied to define the geotechnical features of theformations that the tunnels pass through. The name, thickness and some of the geotechnical properties of the layers are summarized in Table 2 (Ayson 2005). Fill layer of 2.5-m thick consists of sand, clay, gravel and some pieces of masonry. The very stiff clay layer of 4 m is grayish green in color, consisting of gravel and sand. The dense sand layer of 5 m is brown at the upper levels and greenish yellow at the lower levels, consisting of clay, silt and mica. Dense sand of 3 m is greenish yellow and consists of mica. The base layer of the tunnel is hard clay, which is dark green, consisting of shell. The underground water table starts at 4.5 m below the surface. The tunnel axis is 14.5 m below the surface, close to the contact between very dense sand and hard clay. This depth isquite uniform in the chainage between 0 + 850 and 0 + 900 m.Surface settlement prediction with finite element modelingPlaxis finite element code for soil and rock analysis is used to predict the surface settlement. First, the right tube is constructed, and then the left tube 100 m behind the right tube is excavated. This is based on the assumption that ground deformations caused by the excavation of the right tube are stabilized before the excavation of the left tube. The finite element mesh is shown in Fig. 2 using 15 stress point triangular elements. The FEM model consists of 1,838 elements and 15,121 nodes. In FE modeling, the Mohr–Coulomb failure criterion is applied.Staged construction is used in the FE model. Excavation of the soil and the construction of the tunnel lining are carried out in different phases. In the first phase, the soil in front of TBM is excavated, and a support pressure of 300 kPa is applied at the tunnel face to prevent failure at the face. In the first phase, TBM is modeled as shell elements. In the second phase, the tunnel lining is constructedusing prefabricated concrete ring segments, which are bolted together within the tunnel boring machine. During the erection of the lining, TBM remains stationary. Once a lining ring has been bolted, excavation is resumed until sufficient soil excavation is carried out for the next lining. The tunnel lining is modeled using volume elements. In the second phase, the lining is activated and TBM shell elements are deactivated.When applying finite element models, volume loss values are usually assumed prior to excavation. In this study, the FEM model is run with the assumption of 0.5, 0.75, 1 and 1.5% volume loss caused by the convergence of the ground into the tunnel after excavation. Figures 3 and 4 show total and vertical deformations after both tubes are constructed. The vertical ground settlement profile after theright tube construction is given in Fig. 5, which is in theshape of a Gaussian curve, and that after construction of both tubes is given in Fig. 6. Figure 7 shows the total deformation vectors.The maximum ground deformations under different volume loss assumptions are summarized in Table 3.Surface settlement prediction with semi-theoretical and analytical methodsSemi-theoretical predictions for short-term maximum settlement are performed using the Gaussian curve approach, which is a classical and conventional method. The settlement parameters used in semi-theoretical estimations and notations are presented in Fig. 8.The theoretical set tlement (Gaussian) curve is presented as in Eq. 1 (O’Reilly and New 1982):)2(max 22i x e S S -= (1)where, S is the theoretical settlement (Gauss error function, normal probability curve), Smax is the maximum short-term (initial, undrained) settlement at the tunnel centerline (m), x is the transverse horizontal distance from the tunnel center line (m), and i is the point of inflexion (m). To determine the shape of a settlement curve, it is necessary to predict i and Smax values.There are several suggested methods for prediction of the point of inflexion (i). Estimation of i value in this studyis based on averages of some empirical approaches given in Eqs. 2–6:where, Z0 is the tunnel axis depth (m), 14.5 m in this study, and R is the radius of tunnel, 3.25 m in this study. Equation 3 was suggested by Glossop (O’Reilly and New 1982) for mostly cohesive grounds; Eq. 4 was suggested by O’Reilly and New (1982) for excavation of cohesive grounds by shielded machines; Eq. 5 was suggested by Schmidt (1969) for excavation of clays by shielded machines; Eq. 6 was suggested by Arioglu (1992) for excavation of all types of soils by shielded machines. As a result, the average i value is estimated to be 6.6 m in this study.There are several suggested empirical methods for the prediction of the maximum surface settlement (Smax).Schmidt suggested a model for the estimation of Smax value for a single tunnel in 1969 as given in Eq. 7 (through Arioglu 1992):where, K is the volume loss (%). Arioglu (1992), based on field data, found a good relationship between K and N (stability ratio) for face-pressurized TBM cases as in Eq. 8:where cn is the natural unit weight of the soil (kN/m3), the weighted averages for all the layers, which is 19 kN/m3 in this study; rS is the total surcharge pressure (kPa), assumed to be 20 kPa in this study; rT is TBM face pressure (kPa), which is 300 kPa in this study; and CU is the undrained cohesion of the soil (kPa), the weightedaverages for all the layers, which is 50 kPa in this study assuming that CU is equal to SU (undrained shear strength of the soil). Allaverages are estimated up to very dense sand, excluding hard clay, since the tunnel axis passes around the contact between very dense sand and hard clay. The model yields 17.1 mm of initial maximum surface settlement.Herzog suggested a model for the estimation of Smax value in 1985 as given in Eq. 9 for a single tunnel and Eq. 10 for twin tunnels (through Arioglu 1992):where, E is the elasticity modulus of formation (kPa), the weighted averages for all the layers, which is 30,000 kPa in this study, and a is the distance between the tunnel axes, which is 14 m in this study. The model yields 49.9 and 58.7 mm of initial maximum surface settlements for the right and the left tube tunnel, which is 100 mm behind the right tube, respectively.There are several analytical models for the prediction of short-term maximum surface settlements for shielded tunneling operations (Lee et al. 1992; Loganathan and Poulos 1998; Chi et al. 2001; Chou and Bobet 2002; Park 2004). The method suggested by Loganathan and Poulos (1998) is used in this study. In this method, a theoretical gapparameter (g) is defined based on physical gap in the void, face losses and workmanship value, and then the gap parameter is incorporated to a closed form solution to predict elastoplastic ground deformations. The undrained gap parameter (g) is estimated by Eq. 12:where Gp is the physical gap representing the geometric clearance between the outer skin of the shield and the liner, is the thickness of the tail shield, d is the clearance required for erection of the liner, U*3D is the equivalent 3D elastoplastic deformation at the tunnel face, and w is a value that takes into account the quality of workmanship.Maximum short-term surface settlement is predicted by theoretical Eq. 13 (Loganathan and Poulos 1998):where, t is undrained Poisson’s ratio, assumed to be of maximum 0.5; g is the gap parameter (m), which is estimated to be 0.0128 m in this study; and x is transversedistance from the tunnel centerline (m) and it is assumed to be 0 m for the maximum surface settlement. The model yields 23.0 mm of undrained maximum surface settlement.Other parameters of settlement such as maximum slope, maximum curvature and so on are not mentioned in this study.Verification of predictions by field measurements and discussionThe results of measurements performed on the surface monitoring points, by Istanbul Metropolitan Municipality, are presented in Table 4 for the left and right tubes. As seen, the average maximum surface settlements are around 9.6 mm for the right tube and 14.4 mm for the left tube, which excavates 100 m behind the right tube. Themaximum surface settlements measured around 15.2 mm for the right tube and 26.3 mm for the left tube. Higher settlements are expected in the left tube since the previous TBM excavation activities on the right tube overlaps the previous deformation. The effect of the left tube excavation on deformations of the right tube is presented in Fig. 9. As seen, after Lovat TBM in the right tube excavates nearby the surface monitoring point 25, maximum surface settlement reaches at around 9 mm; however, while Herrenknecht TBM in the left tube passes the same point, maximum surface settlement reaches at around 29 mm (Fig. 10).If the construction method applied to the site is considered, long-term (consolidation) settlements are expected to be low, since the tail void is grouted immediately after excavation. The results of predictions mentioned above and observed maximum surface settlements are summarized in Table 5. The methods suggested by Loganathan and Poulos (1998) and Schmidt (1969) connected with Arioglu’s suggestion (1992) can predict the maximum short-term surface settlements only for a single tunnel. Plaxis finite element and Herzog (1985) models can predict deformations for twin tubes.Herzog’s model (1985) yields higher maximum surface settlements than the observed ones. The reason for that is that the database of the model includes both shielded tunnels and NATM (New Austrian Tunneling Method) tunnels, of which surfacesettlements are usually higher compared to shielded tunnels. Schmidt (1969), along withArioglu’s suggestion (1992), yields predictions close to observed.Plaxis finite element modeling gives the most realistic results, provided there is correct assumption of volume loss parameter, which is usually difficult to predict. The model provides simulation of excavation, lining, grouting and face pressure in a realistic manner to predict surface and sub-surface settlements. The volume loss parameter is usually assumed to be \1% for excavation with facepressure-balanced tunnel boring machines. The realized volume loss in the site is around 1% for this study.Currently, there is difficulty yet in modeling the deformation behavior of twin tunnels. One of the most impressive studies on this issue was performed by Chapman et al. (2004). However, Chapman’s semi-theoretical method still requires enlargement of the database to improve the suggested model in his paper.ConclusionsIn this study, three surface settlement prediction methods for mechanized twin tunnel excavations between Esenler and Kirazlı stations of I stanbul Metro Line are applied. Tunnels of 6.5-m diameters with 14-m distance between their centers are excavated by EPM tunnel boring machines. The geologic structure of the area can be classified as soft ground.Settlement predictions are performed by using FE modeling, and semi-theoretical (semi-empirical) and analytical methods. The measured results after tunneling are compared to predicted results. These indicate that the FE model predicts well the short time surface settlements for a given volume loss value. The results of some semi-theoretical and analytical methods are found to be in goodagreement with the FE model, whereas some methods overestimate the measured settlements. The FE model predicted the maximum surface settlement as 15.89 mm (1% volume loss) for the right tube, while the measured maximum settlement was 15.20 mm. For the left tube (opened after the right), FE prediction was 24.34 mm, while measured maximum settlement was 26.30 mm.中文翻译基于盾构法的Istanbul地铁施工引起的地面沉降预测摘要在这项研究中,研究的是双线隧道的短期地面沉降,选取线路里程总长为4km的Istanbul地铁从Esenler站到Kirazl站方向850到900m区间为研究对象。
城市地下空间工程中英文对照外文翻译文献
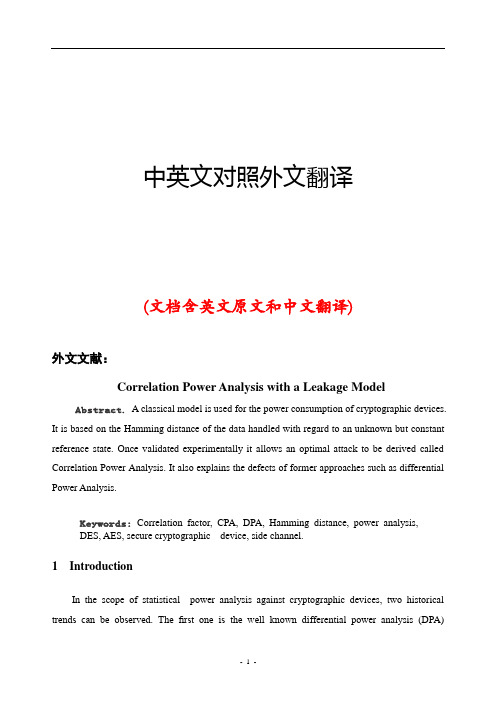
中英文对照外文翻译(文档含英文原文和中文翻译)外文文献:Correlation Power Analysis with a Leakage Model Abstract.A classical model is used for the power consumption of cryptographic devices. It is based on the Hamming distance of the data handled with regard to an unknown but constant reference state. Once validated experimentally it allows an optimal attack to be derived called Correlation Power Analysis. It also explains the defects of former approaches such as differential Power Analysis.Keywords:Correlation factor, CPA, DPA, Hamming distance, power analysis,DES, AES, secure cryptographic device, side channel.1 IntroductionIn the scope of statistical power analysis against cryptographic devices, two historical trends can be observed. The first one is the well known di fferential power analysis (DPA)introduced by Paul Kocher [12,13] and formalized by Thomas Messerges etal. [16]. The second one has been suggested in various papers [8,14,18] and proposed to use the correlation factor between the power samples and the Hamming weight of the handled data. Both approaches exhibit some limitations due to unrealistic assumptions and model imperfections that will be examined more thoroughly in this paper. This work follows previous studies aiming at either improving the Hamming weight model [2], or enhancing the DPA itself by various means [6,4].The proposed approach is based on the Hamming distance model which can be seen as a generalization of the Hamming weight model. All its basic assumptions were already mentioned in various papers from year 2000 [16,8,6,2]. But they remained allusive as possible explanation of DPA defects and never leaded to any complete and convenient exploitation. Our experimental work is a synthesis of those former approaches in order to give a full insight on the data leakage. Following [8,14,18] we propose to use the correlation power analysis (CPA) to identify the parameters of the leakage model. Then we show that sound and efficient attacks can be conducted against unprotected implementations of many algorithms such as DES or AES. This study deliberately restricts itself to the scope of secret key cryptography although it may be extended beyond.This paper is organized as follows: Section 2 introduces the Hamming distance model and Section 3 proves the relevance of the correlation factor. The model based correlation attack is described in Section 4 with the impact on the model errors. Section 5 addresses the estimation problem and the experimental results which validate the model are exposed in Section 6. Section 7 contains the comparative study with DPA and addresses more specifically the so-called “ghost peaks” problem encountered by those who have to deal with erroneous conclusions when implementing classical DPA on the substitution boxes of the DES first round: it is shown there how the proposed model explains many defects of the DPA and how the correlation power analysis can help in conducting sound attacks in optimal conditions. Our conclusion summarizes the advantages and drawbacks of CPA versus DPA and reminds that countermeasures work against both methods as well.2 The Hamming Distance Consumption ModelClassically, most power analyses found in literature are based upon the Hamming weight model [13,16], that is the number of bits set in a data word. In a m-bit microprocessor, binary data is coded ∑-==102m j j j d D , with the bit values dj=0 or 1. Its Hamming weight is simply the number of bits set to 1,∑-==10)(m j j d D H Its integer values stand between 0 and m. If D contains m independent and uniformly distributed bits, the whole word has an average Hammingweight 2/m H =μand a variance 4/2m H =σ. It is generally assumed that the data leakage through the power side-channel depends on the number of bits switching from one state to the other [6,8] at a given time. A microprocessor is modeled as a state where transitions from state to state are triggered by events such as the edges of a clock signal.This seems relevant when looking at a logical elementary gate as implemented in CMOS technology. The current consumed is related to the energy required to flip the bits from one state to the next. It is composed of two main contributions: the capacitor’s charge and the short circuit induced by the gate transition. Curiously,this elementary behavior is commonly admitted but has never given rise to any satisfactory model that is widely applicable. Only hardware designers are familiar with simulation tools to foresee the current consumption of microelectronic devices.If the transition model is adopted, a basic question is posed: what is the reference state from which the bits are switched? We assume here that this reference state is a constant machine word, R, which is unknown, but not necessarily zero. It will always be the same if the same data manipulation always occurs at the same time, although this assumes the absence of any desyn chronizing effect.Moreover, it is assumed that switching a bit from 0 to 1 or from 1 to 0 requires the same amount of energy and that all the machine bits handled at a given time are perfectly balanced and consume the same.These restrictive assumptions are quite realistic and affordable without any thorough knowledge of microelectronic devices. They lead to a convenient expression for the leakage model. Indeed the number of flipping bits to go from R to D is described by H (D ⊕R) also called the Hamming distance between D and R. This statement encloses the Hamming weight modelb which assumes that R = 0. If D is a uniform random variable, so is D ⊕R, and H (D ⊕R) has the same mean m/2 and variance m/4 as H (D).We also assume a linear relationship between the current consumption and H (D ⊕R). This can be seen as a limitation but considering a chip as a large set of elementary electrical components, this linear model fits reality quite well. It does not represent the entire consumption of a chip but only the data dependent part. This does not seem unrealistic because the bus lines are usually considered as the most consuming elements within a micro-controller. All the remaining things in the power consumption of a chip are assigned to a term denoted b which is assumed independent from the other variables: encloses of sets, time dependent components and noise. Therefore the basic model for the data dependency can be written:b R D aH W +⊕=)(where a is a scalar gain between the Hamming distance and W the power consumed.3 The Linear Correlation FactorA linear model implies some relationships between the variances of the different terms considered as random variables:2222b H W a σσσ+=,Classical statistics introduce the correlationfactor ρWH between the Hamming distance and the measured power to assess the linear modelfitting rate. It is the covariance between both random variables normalized by the product of their standard deviations.Under the uncorrelated noise assumption, this definition leads to:222224),cov(b b b H H W WH ma m a a a H W σσσσσσρ+=+==This equation complies with the well known property: −1 ≤ ρWH ≤ +1: for a perfect model the correlation factor tends to ±1 if the variance of noise tends to0, the sign depending on the sign of the linear gain a. If the model applies only to l independent bits amongst m, a partial correlation still exists:ml ma la H WHb m l W ρσρ=+=22/44 Secret Inference Based on Correlation Power AnalysisThe relationships written above show that if the model is valid the correlation factor is maximized when the noise variance is minimum. This means that ρWH can help to determine the reference state R. Assume, just like in DPA, that a set of known but randomly varying data D and a set of related power consumption W are available. If the 2m possible values of R are scanned exhaustively they can be ranked by the correlation factor they produce when combined with the observation W . This is not that expensive when considering an 8-bit micro- controller, the case with many of today’s smart cards, as only 256 values are to be tested. On 32-bit architectures this exhaustive search cannot be applied as such. But it is still possible to work with partial correlation or to introduce prior knowledge.Let R be the true reference and H = H (D ⊕R) the right prediction on the Hamming distance. Let R represent a candidate value and H the related model H = H (D ⊕ R ). Assume a value of R that has k bits that differ from those of R, then: H (R ⊕ R ) = k. Since b is independent from other variables, the correlation test leads to (see [5]):mk m H H a H b aH WH HH WH H W H W WH 2)',cov()',cov(''''-===+=ρρρσσσσρ This formula shows how the correlation factor is capable of rejecting wrong candidates for R. For instance, if a single bit is wrong amongst an 8-bit word, the correlation is reduced by 1/4. If all the bits are wrong, i-e R = ¬R, then an anti-correlation should be observed with ρWH = −ρWH . In absolute value or if the linear gain is assumed positive (a > 0), there cannot be any R leading to a higher correlation rate than R. This proves the uniqueness of the solution and therefore how the reference state can be determined.This analysis can be performed on the power trace assigned to a piece of code while manipulating known and varying data. If we assume that the handled data is the result of a XOR operation between a secret key word K and a known message word M , D = K ⊕ M , the procedure described above, i-e exhaustive search on R and correlation test, should lead to K ⊕ R as sociated with max(ρWH ). Indeed if a correlation occurs when M is handled with respect to R1 , another has to occur later on, when M ⊕ K is manipulated in turn, possibly with a different reference state R2 (in fact with K ⊕ R2 since only M is known).For instance, when considering the first Add Round Key function at the beginning of the AES algorithm embedded on an 8-bit processor, it is obvious that such a method leads to the whole key masked by the constant reference byte R2 . If R2 is the same for all the key bytes, which is highly plausible, only 28 possibilities remain to be tested by exhaustive search to infer the entire key material. This complementary brute force may be avoided if R2 is determined by other means or known to be always equal to 0 (on certain chips).This attack is not restricted to the ⊕ operation. It also applies to many other operators often encountered in secret key cryptography. For instance, other arithmetic, logical operations or look-up tables (LUT) can be treated in the same manner by using H(LUT(M K ) ⊕ R), where represents the involved function i.e. ⊕, +, -, OR, AND, or whatever operation. Let’s notice that the ambiguity between K and K ⊕R is completely removed by the substitution boxes encountered in secret key algorithms thanks to the non-linearity of the corresponding LUT: this may require to exhaust both K and R, but only once for R in most cases. To conduct an analysis in the best conditions, we emphasize the benefit of correctly modeling the whole machine word that is actually handled and its transition with respect to the reference state R which is to be determined as an unknown of the problem.5 EstimationIn a real case with a set of N power curves Wi and N associated random data words Mi , for a given reference state R the known data words produce a set of N predicted Hamming distances Hi,R = H (Mi ⊕ R). An estimate ρˆWH of the correlation factor ρWH is given by the following formula:∑∑∑∑∑∑∑---=2,2,22,,^)()()(R i R i i i Ri i R i i WH H H N W W N H W H W N R ρwhere the summations are taken over the N samples (i = 1,N ) at each time step within the power traces Wi (t).It is theoretically diffi cult to compute the variance of the estimator ρˆWH with respect to the number of available samples N . In practice a few hundred experiments suffice to provide a workable estimate of the correlation factor. N has to be increased with the model variance m/4 (higher on a 32-bit architecture) and in presence of measurement noise level obviously. Next results will show that this is more than necessary for conducting reliable tests. The reader is referred to [5] for further discussion about the estimation on experimental data and optimalityissues. It is shown that this approach can be seen as a maximum likelihood model fitting procedure when R is exhausted to maximize ρˆWH .6 Experimental ResultsThis section aims at confronting the leakage model to real experiments. General rules of behavior are derived from the analysis of various chips for secure devices conducted during the passed years.Our first experience was performed onto a basic XOR algorithm impl emented in a 8-bit chip known for leaking information (more suitable for didactic purpose). The sequence of instructions was simply the following:–load a byte D1 into the accumulator–XOR D1 with a constant D2–store the result from the accumulator to a destination memory cell.The program was executed 256 times with D1 varying from 0 to 255. As displayed on Figure 1, two significant correlation peaks were obtained with two di fferent reference states: the first one being the address of D1 , the second one the op code of the XOR instruction. These curves bring the experimental evidence of leakage principles that previous works just hint at, without going into more detail [16,8,6,17]. They illustrate the most general case of a transfer sequence on a common bus. The address of a data word is transmitted just before its value that is in turn immediately followed by the op code of the next instruction which is fetched. Such a behavior can be observed on a wide variety of chips even those implementing 16 or 32-bit architectures. Correlation rates ranging from 60% to more than 90% can often be obtained. Figure 2 shows an example of partial correlation on a 32-bit architecture: when only 4 bits are predicted among 32, the correlation loss is in about the ratio √8 which is consistent with the displayed correlations.This sort of results can be observed on various technologies and implementations. Nevertheless the following restrictions have to be mentioned:–Sometimes the reference state is systematically 0. This can be assigned to the so-calledcharged logic where the bus is cleared between each transferred value. Another possible reason is that complex architectures implement separated for data and addresses, that may prohibit certain transitions. In all those cases the Hamming weight model is recovered as a particular case of the more general Hamming distance model.–The sequence of correlation peaks may sometimes be blurred or spread over the time in presence of a pipe line.–Some recent technologies implement hardware security features designed to impede statistical power analysis. These countermeasures offer various level so going from the most naive and easy to bypass, to the most effective which merely cancel any data dependency.There are different kinds of countermeasures which are completely similar to those designed against DPA.–Some of them consist in introducing desyn chronization in the execution of the process so that the curves are not aligned anymore within a same acquisition set. For that purpose there exist various techniques such as fake cycles insertion, unstable clocking or random delays [6,18]. In certain cases their effect can be corrected by applying appropriate signal processing.Fig. 1. Upper: consecutive correlation peaks for two different reference states.Lower: for varying data (0-255), model array and measurement array taken at the time of the secondcorrelation peak.Fig. 2. Two correlation peaks for full word (32 bits) and partial (4 bits) predictions. According to theorythe 20% peak should rather be around 26%.–Other countermeasures consist in blurring the power traces with additional noise or filtering circuitry [19]. Sometimes they can be bypassed by curves selection and/or averaging or by using another side channel such as electromagnetic radiation [9,1].–The data can also be ciphered dynamically during a process by hardware(such as bus encryption) or software means (data masking with a random[11,7,20,10]), so that the handled variables become unpredictable: then no correlation can be expected anymore. In theory sophisticated attacks such as higher order analysis [15] can overcome the data masking method; but they are easy to thwart in practice by using desyn chronization for instance.Indeed, if implemented alone, none of these countermeasures can be considered as absolutely secure against statistical analyses. They just increase the amount of effort and level of expertise required to achieve an attack. However combined defenses, implementing at least two of these countermeasures, prove to be very efficient and practically dissuasive. The state of the art of countermeasures in the design of tamper resistant devices has made big advances in the recent years.It is now admitted that security requirements include sound implementations as much as robust cryptographic schemes.7 Comparison with DPAThis section addresses the comparison of the proposed CPA method with Differential Power Analysis (DPA). It refers to the former works done by Messerges etal. [16,17] who formalized the ideas previously suggested by Kocher [12,13]. A critical study is proposed in [5].7.1 Practical Proble ms with DPA: The “Ghost Peaks”We just consider hereafter the practical implementation of DPA against the DES substitutions (1st round). In fact this well-known attack works quite well only if the following assumptions are fulfilled:1. Word space assumption: within the word hosting the predicted bit, the contribution of the non-targeted bits is independent of the targeted bit va lue. Their average influence in the curves pack of 0 is the same as that in the curves pack of 1. So the attacker does not need to care about these bits.2. Guess space assumption: the predicted value of the targeted bit for any wrong sub-key guess does not depend on the value associated to the correct guess.3. Time space assumption: the power consumption W does not depend on the value of the targeted bit except when it is explicitly handled.But when confronted to the experience, the attack comes up against the following facts.–Fact A. For the correct guess, DPA peaks appear also when the targeted bit is not explicitly handled. This is worth being noticed albeit not really embarrassing. However this contradicts the third assumption.–Fact B. Some DPA peaks also appear for wrong guesses: they are called “ghost peaks”. This fact is more problematic for making a sound decision and comes in contradiction with the second assumption.–Fact C. The true DPA peak given by the right guess may be smaller than some ghost peaks, and even null or negative! This seems somewhat amazing and quite confusing for an attacker. The reasons must be searched for inside the crudeness of the optimistic first assumption.7.2 The “Ghost Peaks” ExplanationWith the help of a thorough analysis of substitution boxes and the Hamming distance model it is now possible to explain the observed facts and show how wrong the basic assumptions of DPA can be.Fact A. As a matter of fact some data handled along the algorithm may be par- tially correlated with the targeted bit. This is not that surprising when looking at the structure of the DES. A bit taken from the output nibble of a S Box has a lifetime lasting at least until the end of the round (and beyond if the left part of the IP output does not vary too much). A DPA peak rises each time this bit and its 3 peer bits undergo the following P permutation since they all belong to the same machine word.Fact B. The reason why wrong guesses may generate DPA peaks is that the distributions of an S Box output bit for two different guesses are deterministic and so possibly partially correlated. The following example is very convincing about that point. Let’s consider the leftmost bit of the fifth S Box of the DES when the input data D varies from 0 to 63 and combined with two different sub-keys : MSB(SBox5 (D ⊕ 0x00)) and MSB(SBox5 (D ⊕ 0x36)). Both series of bits are respectively listed hereafter, with their bit wise XOR on the third line: 110110101001011000100101100100111010100101101101010100100010110110011010110101100010010111010010101011010110100101010010001110010100000001000000000000000100000100000100000001000000000000010100The third line contains 8 set bits, revealing only eight errors of prediction among 64. This example shows that a wrong guess, say 0, can provide a good prediction at a rate of 56/64, that is not that far from the correct one 0x36. The result would be equivalent for any other pair of sub-keys K and K ⊕ 0x36. Consequently a substantial concurrent DPA peak will appear at the same location than the right one. The weakness of the contrast will disturb the guesses ranking especially in presence of high SN R.Fact C. DPA implicitly considers the word bits carried along with the targeted bit as uniformly distributed and independent from the targeted one. This is erroneous because implementation introduces a deterministic link between their values. Their asymmetric contribution may aff ect the height and sign of a DPA peak. This may influence the analysis onthe one hand by shrinking relevant peaks, on the other hand by enhancing meaningless ones. There exists a well known trick to bypass this difficulty as mentioned in [4]. It consists in shifting the DPA attacks a little bit further in the processing and perform the prediction just after the end of the first round when the right part of the data (32 bits) is XO Red with the left part of the IP output. As the message is chosen freely, this represents an opportunity to re-balance the loss of randomness by bringing new refreshed random data. But this does not fix Fact B in a general case .To get rid of these ambiguities the model based approach aims at taking the whole information into account. This requires to introduce the notion of algorithmic implementation that DPA assumptions completely occult.When considering the substitution boxes of the DES, it cannot be avoided to remind that the output values are 4-bit values. Although these 4 bits are in principle equivalent as DPA selection bits, they live together with 4 other bits in the context of an 8-bit microprocessor. Efficient implementations use to exploit those 4 bits to save some storage space in constrained environments like smart c ard chips. A trick referred to as “SBox compression” consists in storing 2 SBox values within a same byte. Thus the required space is halved. There are different ways to implement this. Let’s consider for instance the 2 first boxes: instead of allocating 2different arrays, it is more efficient to build up the following look- up table: LUT12 (k) = SBox1 (k) SBox2 (k). For a given input index k, the array byte contains the values of two neighboring boxes. Then according to the Hamming distance consumption model, the power trace should vary like:–H(LUT12 (D1 ⊕ K1 ) ⊕ R1 ) when computing SBox1 .–H(LUT12 (D2 ⊕ K2 ) ⊕ R2 ) when computing SBox2 .If the values are bind like this, their respective bits cannot be considered as independent anymore. To prove this assertion we have conducted an experiment on a real 8-bit implementation that was not protected by any DPA countermeasures. Working in a “white box” mode, the model parameters had been previously calibrated with respect to the measured consumptio n traces. The reference state R = 0xB7 had been identified as the Op code of an instruction transferring the content of the accumulator to RAM using direct addressing. Themodel fitted the experimental data samples quite well; their correlation factor even r eached 97%. So we were able to simulate the real consumption of the Sbox output with a high accuracy. Then the study consisted in applying a classical single bit DPA to the output of SBox1 in parallel on both sets of 200 data samples: the measured and the simulated power consumptions.As figure 3 shows, the simulated and experimental DPA biases match particularly well. One can notice the following points:–The 4 output bits are far from being equivalent.–The polarity of the peak associated to the correct guess 24 depends on the polarity of the reference state. As R = 0xB7 its leftmost nibble aligned with SBox1 is 0xB = ’1011’ and only the selection bit 2 (counted from the left) results in a positive peak whereas the 3 others undergo a transition from 1 to 0, leading to a negative peak.–In addition this bit is a somewhat lucky bit because when it is used as selection bit only guess 50 competes with the right sub-key. This is a particular favorable case occurring here on SBox1 , partly due to the set of 200 used messages. It cannot be extrapolated to other boxes.–The dispersion of the DPA bias over the guesses is quite confuse (see bit 4).The quality of the modeling proves that those facts cannot be incriminated to the number of acquisitions. Increasing it much higher than 200 does not help: the level of the peaks with respect to the guesses does not evolve and converges to the same ranking. This particular counter-example proves that the ambiguity of DPA does not lie in imperfect estimation but in wrong basic hypotheses.Fig. 3. DPA biases on SBox1 versus guesses for selection bits 1, 2, 3 and 4, on modeled and experimental data; the correct guess is 24.7.3 Results of Model Based CPAFor comparison the table hereafter provides the ranking of the 6 first guesses sorted by decreasing correlation rates. This result is obtained with as few as only 40 curves! The full key is 11 22 33 44 55 66 77 88 in hexadecimal format and the corresponding sub-keys at the first round are 24, 19, 8, 8, 5, 50, 43, 2 in decimal representation.SBox1 SBox2SBox3SBox4SBox5SBox6SBox7SBox8K ρmax K ρmax K ρmax K ρmax K ρmax K ρmax K ρmax K ρmax24 92%19 90%887%888%591%50 92%43 89% 289%48 74%18 77%18 69%44 67%32 71%25 71%42 76%28 77% 01 74%57 70%05 68%49 67%25 70%05 70%52 70%61 76%This table shows that the correct guess always stands out with a good contrast. Therefore a s ound decision can be made without any ambiguity despite a rough estimation of ρmax .A similar attack has also been conducted on a 32-bit implementation, in a white box mode with a perfect knowledge of the implemented substitution tables and the reference state which was 0. The key was 7C A1 10 45 4A 1A 6E 57 in hexadecimal format and the related sub-keys at the 1st round were 28, 12, 43,0, 15, 60, 5, 38 in decimal representation. The number of curves is 100. As next table shows, the contrast is good between the correct and the most competing wrong guess (around 40% on boxes 1 to 4). The correlation rate is not that high on boxes 5 to 8, definitely because of partial and imperfect modeling, but it proves to remain exploitable and thus a robust indicator. When the number of bits per machine word is greater, the contrast between the guesses is relatively enhanced, but finding the right model could be more difficult in a black box mode.8 ConclusionOur experience on a large set of smart card chips over the last years has convinced us on the validity of the Hamming distance model and the advantages of the CPA method against DPA, in terms of efficiency, robustness and number of experiments. An important and reassuring conclusion is that all the countermeasures designed against DPA offer the same defensiveefficiency against the model based CPA attack. This is not that surprising since those countermeasures aim at undermining the common prerequisites that both approaches are based on: side-channel observability and intermediate variable predictability.The main drawback of CPA regards the characterization of the leakage model parameters. As it is more demanding than DPA, the method may seem more difficult to implement. However it may be objected that:– A statistical power analysis of any kind is never conducted blindly without any preliminary reverse engineering (process identification, bit tracing): this is the opportunity to quantify the leakage rate by CPA on known data.–DPA requires more sample curves anyway since all the predicted data bits penalize the signal to noise ratio (see [5]).–If DPA fails by lack of implementation knowledge (increasing the number of curves does not necessarily help), we have shown how to infer a part of this information without excessive efforts: for instance the reference state is to be found by exhaustive search only once in general.–There exists many situations where the implementation variants (like SBox implementation in DES) are not so numerous because of operational constraints.–If part of the model cannot be inferred (SBox implementation in DES, hardware co-processor), partial correlation with the remainder may still provide exploitable indications.Eventually DPA remains relevant in case of very special architectures for which the model may be completely out of reach, like in certain hard wired co- processors.References1. D. Agrawal, B. Archambeault, J.R. Rao, and P. Rohatgi. The EM side channel(s): Attacks and assessment methodologies. In Cryptographic Hardware and Embedded Systems —CHES2002,LNCS2523,pp29–45,Springer-Verlag,2002.Seealso.ints ec/emf-paper.ps.2. M.L. Akkar, R. B´evan, P. Dischamp, and D. Moyart. Power analysis, what is now possible... In Advances in Cryptology —ASIACRYPT 2000, LNCS 1976, pp.489–502,。
城市轨道交通-专业术语(中英文对照)

地铁 metro/underground railway/subway城市轨道交通 urban rail transit/mass transit设计使用年限 designed lifetime运营概念 operation concept旅行速度 operation speed限界 gauge 轨道结构 track structure正线 main line 辅助线 assistant line 联络线 connecting line 试车线 testing line轨距 gauge of track无缝线路 seamless track整体道床 monolithic track-bed路基 subgrade站台计算长度 computed length of platform车站公共区 public zone of station无缝线路纵向水平力 longitudinal horizontal force of seamless track无缝线路断轨力 broken rail force of seamless track明挖法 cut and cover盖挖顺筑法 cover and cut—bottom up盖挖逆筑法 cover and cut-top down矿山法 mining method盾构法 shield method沉管法 immersed tube method防水等级 grade of waterproof变形缝 deformation joint刚柔结合的密封区 rigid—flexible joint of sealed zone开式运行 open made operation闭式运行 close made operation活塞通风 piston action ventilation合流制排放 combined sewer system集中式供电 centralized power supply mode分散式供电 distribute power supply mode混合式供电 combined power supply mode主变电所 high voltage substation牵引降压混合变电所 combined substation杂散电流 stray current同步数字传输系统 synchronous digital hierarchy transmission system(SDH)全球定位系统 global position system(GPS)列车自动控制automatic train control(ATC)列车自动监控 automatic train supervision(ATS)列车自动防护automatic train protection(ATP)列车自动运行 automatic train operation(ATO)调度集中 centralized traffic control(CTC)自动人行道 moving pavement自动售检票设备 automatic fare collection火灾自动报警系统 fire alarm system区域报警系统 local alarm system集中报警系统 remote alarm system环境与设备监控系统 building automatic system(BAS)系统集成system integration(SI)运营控制中心operation control center(OCC)集中监控和管理 concentration supervisory control and management 车辆段 depot停车场 stabling yard检修修程 examine and repair program检修周期 examine and repair period建筑设备自动化系统 Building Automation System供电系统管理自动化 Scan Control Alarm Database人机接口 Man Machine Interface不间断电源供给 Uninterrupted Power Supply南京一卡通系统 Nanjing Transportation Card System建设部 Ministry of Corporation南京地铁公司 Nanjing Metro Corporation清结算数据中心 Intermodality Data Center局域网 Local Area Network广域网 Wide Area Network开放传输网络 Open Transport Network拖车 Trailer Car(Tc(A))带受电弓的动车 Motor Car With Pantograph(Mp(B))动车 Motor Car(M(C))空载 AW0每位乘客都有座位 AW1每平方米6人 AW2每平方米9人 AW3非接触智能卡 Contactless Smart Card(CSC)非接触智能筹码 Contactless Smart Card(CST)设备运行参数 Equipment Operating Data(EOD)。
城市沉降 英语
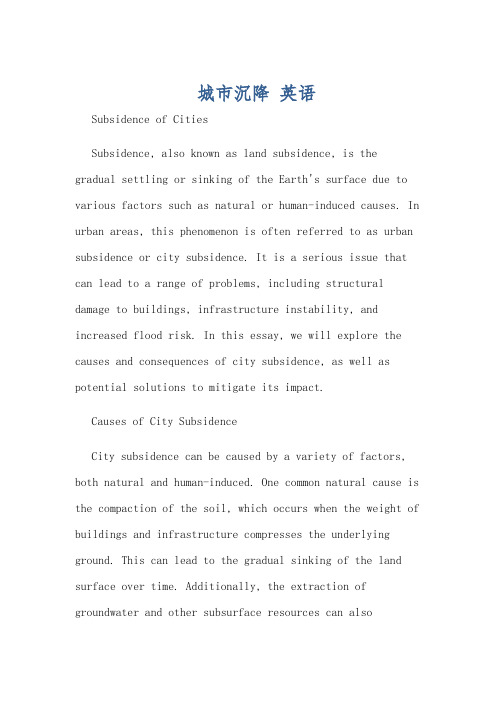
城市沉降英语Subsidence of CitiesSubsidence, also known as land subsidence, is the gradual settling or sinking of the Earth's surface due to various factors such as natural or human-induced causes. In urban areas, this phenomenon is often referred to as urban subsidence or city subsidence. It is a serious issue that can lead to a range of problems, including structural damage to buildings, infrastructure instability, and increased flood risk. In this essay, we will explore the causes and consequences of city subsidence, as well as potential solutions to mitigate its impact.Causes of City SubsidenceCity subsidence can be caused by a variety of factors, both natural and human-induced. One common natural cause is the compaction of the soil, which occurs when the weight of buildings and infrastructure compresses the underlying ground. This can lead to the gradual sinking of the land surface over time. Additionally, the extraction of groundwater and other subsurface resources can alsocontribute to subsidence, as this process can lead to the collapse of underground voids and the compaction of the surrounding soil.Human-induced causes of city subsidence include the excessive extraction of groundwater for agricultural, industrial, and domestic use. When large volumes of water are pumped from underground aquifers, the surrounding soil can compact and settle, leading to subsidence. In some cases, the construction of underground tunnels and mining activities can also contribute to city subsidence by destabilizing the ground and causing it to sink.Consequences of City SubsidenceThe consequences of city subsidence can be significant and wide-ranging. One of the most immediate and visible impacts is the damage to buildings and infrastructure. As the ground sinks, structures may experience uneven settlement, leading to cracks in walls, floors, and foundations. This can compromise the structural integrity of buildings and pose a safety risk to occupants. In addition, subsidence can also cause damage to infrastructure such as roads, bridges, and undergroundutilities, leading to costly repairs and disruptions to transportation networks.Another consequence of city subsidence is the increased risk of flooding. When the land sinks, drainage systems become less effective at carrying away surface water, leading to water pooling and increased flood risk, particularly during heavy rainfall events. This can have serious implications for public safety and property damage, as well as economic and social disruption.Solutions to Mitigate City SubsidenceAddressing city subsidence requires a multi-faceted approach that includes both preventive measures and adaptive strategies. One key preventive measure is the sustainable management of groundwater resources. This involves implementing regulations and policies to limit excessive groundwater extraction and promote the use of alternative water sources, such as recycled water and rainwater harvesting.In addition, urban planning and land use regulations can play a crucial role in mitigating city subsidence. By carefully managing the location and density of development,as well as enforcing building codes that consider subsidence risk, cities can reduce the potential for structural damage and infrastructure instability. Furthermore, the use of innovative engineering techniques, such as ground reinforcement and soil stabilization, can help to mitigate the effects of subsidence and protect buildings and infrastructure from damage.Finally, ongoing monitoring and early warning systemsare essential for detecting and responding to city subsidence. By regularly surveying land surface elevation and subsurface conditions, cities can identify areas atrisk of subsidence and take proactive measures to address the issue before it escalates. Additionally, public education and awareness campaigns can help to inform residents and businesses about the risks of subsidence and the importance of sustainable land use practices.ConclusionCity subsidence is a complex and challenging problemthat requires coordinated efforts from government, industry, and the public to address. By understanding the causes and consequences of subsidence, and implementing proactive andsustainable solutions, cities can mitigate the impact of subsidence and ensure the long-term stability andresilience of urban areas.城市沉降城市沉降,也称为土地沉降,是地表逐渐下沉或下沉的现象,由于各种因素,如自然或人为原因。
土木外文翻译原文和译文

A convection-conduction model for analysis of thefreeze-thawconditions in the surrounding rock wall of atunnel in permafrost regionsAbstractBased on the analyses of fundamental meteorological and hydrogeological conditions at the site of a tunnel in the cold regions, a combined convection-conduction model for air flow in the tunnel and temperature field in the surrounding has been constructed. Using the model, the air temperature distribution in the Xiluoqi No. 2 Tunnel has been simulated numerically. The simulated results are in agreement with the data observed. Then, based on the in situ conditions of sir temperature, atmospheric pressure, wind force, hydrogeology and engineering geology, the air-temperature relationship between the temperature on the surface of the tunnel wall and the air temperature at the entry and exit of the tunnel has been obtained, and the freeze-thaw conditions at the Dabanshan Tunnel which is now under construction is predicted.Keywords: tunnel in cold regions, convective heat exchange and conduction, freeze-thaw.A number of highway and railway tunnels have been constructed in the permafrost regions and their neighboring areas in China. Since the hydrological and thermal conditions changed after a tunnel was excavated,the surrounding wall rock materials often froze, the frost heaving caused damage to the liner layers and seeping water froze into ice diamonds,which seriously interfered with the communication and transportation. Similar problems of the freezing damage in the tunnelsalso appeared in other countries like Russia, Norway and Japan .Hence it is urgent to predict the freeze-thaw conditions in the surrounding rock materials and provide a basis for the design,construction and maintenance of new tunnels in cold regions.Many tunnels,constructed in cold regions or their neighbouring areas,pass through the part beneath the permafrost base .After a tunnel is excavated,the original thermodynamical conditions in the surroundings are and thaw destroyed and replaced mainly by the air connections without the heat radiation, the conditions determined principally by the temperature and velocity of air flow in the tunnel,the coefficients of convective heat transfer on the tunnel wall,and the geothermal heat. In order to analyze and predict the freeze and thaw conditions of the surrounding wall rock of a tunnel,presuming the axial variations of air flow temperature and the coefficients of convective heat transfer, Lunardini discussed the freeze and thaw conditions by the approximate formulae obtained by Sham-sundar in study of freezing outside a circular tube with axial variations of coolant temperature .We simulated the temperature conditions on the surface of a tunnel wall varying similarly to the periodic changes of the outside air temperature .In fact,the temperatures of the air and the surrounding wall rock material affect each other so we cannot find the temperature variations of the air flow in advance; furthermore,it is difficult to quantify the coefficient of convective heat exchange at the surface of the tunnel wall .Therefore it is not practicable to define the temperature on the surface of the tunnel wall according to the outside air temperature .In this paper, we combine the air flow convective heat ex-change and heat conduction in the surrounding rock material into one model,and simulate the freeze-thaw conditions of the surrounding rock material based on the in situ conditions of air temperature,atmospheric pressure,wind force at the entry and exit of the tunnel,and the conditions of hydrogeology and engineering geology.Mathematical modelIn order to construct an appropriate model, we need the in situ fundamental conditions as a ba-sis .Here we use the conditions at the scene of the Dabanshan Tunnel. The Dabanshan Tunnel is lo-toted on the highway from Xining to Zhangye, south of the Datong River, at an elevation of 3754.78-3 801.23 m, with a length of 1 530 m and an alignment from southwest to northeast. The tunnel runs from the southwest to the northeast.Since the monthly-average air temperature is beneath 0`}C for eight months at the tunnel site each year and the construction would last for several years,the surrounding rock materials would become cooler during the construction .We conclude that, after excavation, the pattern of air flow would depend mainly on the dominant wind speed at the entry and exit,and the effects of the temperature difference between the inside and outside of the tunnel would be very small .Since the dominant wind direction is northeast at the tunnel site in winter, the air flow in the tunnel would go from the exit to the entry. Even though the dominant wind trend is southeastly in summer, considering the pressure difference, the temperature difference and the topography of the entry and exit,the air flow in the tunnel would also be from the exit to entry .Additionally,since the wind speed at the tunnel site is low,we could consider that the air flow would be principally laminar.Based on the reasons mentioned,we simplify the tunnel to a round tube,and consider that theair flow and temperature are symmetrical about the axis of the tunnel,Ignoring the influence of the air temperature on the speed of air flow, we obtain the following equation:where t ,x ,r are the time ,axial and radial coordinates; U ,V are axial and radial wind speeds; T is temperature; p is the effective pressure(that is ,air pressure divided by air density); v is the kinematic viscosity of air; a is the thermal conductivity of air; L is the length of the tunnel; R is the equivalent radius of the tunnel section; D is the length of time after the tunnel construction;,f S (t), u S (t) are frozen and thawed parts in the surrounding rock materials respectively; f λ,u λand f C ,u C are thermal conductivities and volumetric thermal capacities in frozen and thawed parts respectively; X= (x , r),ξ(t) is phase change front; Lh is heat latent of freezing water; and To is critical freezing temperature of rock ( here we assume To= -0.1℃).2 used for solving the modelEquation(1)shows flow. We first solve those concerning temperature at that the temperature of the surrounding rock does not affect the speed of air equations concerning the speed of air flow, and then solve those equations every time elapse.2. 1 Procedure used for solving the continuity and momentum equationsSince the first three equations in(1) are not independent we derive the second equation by xand the third equation by r. After preliminary calculation we obtain the following elliptic equation concerning the effective pressure p:Then we solve equations in(1) using the following procedures:(i ) Assume the values for U0,V0;( ii ) substituting U0,V0 into eq. (2),and solving (2),we obtain p0;(iii) solving the first and second equations of(1),we obtain U0,V1;(iv) solving the first and third equations of(1),we obtain U2,V2; (v) calculating the momentum-average of U1,v1 and U2,v2,we obtain the new U0,V0;then return to (ii);(vi) iterating as above until the disparity of those solutions in two consecutive iterations is sufficiently small or is satisfied,we then take those values of p0,U0 and V0 as the initial values for the next elapse and solve those equations concerning the temperature..2 .2 Entire method used for solving the energy equationsAs mentioned previously,the temperature field of the surrounding rock and the air flow affect each other. Thus the surface of the tunnel wall is both the boundary of the temperature field in the surrounding rock and the boundary of the temperature field in air flow .Therefore, it is difficult to separately identify the temperature on the tunnel wall surface,and we cannot independently solve those equations concerning the temperature of air flow and those equations concerning the temperature of the surrounding rock .In order to cope with this problem,we simultaneously solve the two groups of equations based on the fact that at the tunnel wall surface both temperatures are equal .We should bearin mind the phase change while solving those equations concerning the temperature of the surrounding rock ,and the convection while solving those equations concerning the temperature of the air flow, and we only need to smooth those relative parameters at the tunnel wall surface .The solving methods for the equations with the phase change are the same as in reference [3].2.3 Determination of thermal parameters and initial and boundaryconditions2.3.1 Determination of the thermal parameters. Using p= 1013.25-0.1088 H ,we calculateair pressure p at elevation H and calculate the air density ρ using formula GTP =ρ, where T is the yearly-average absolute air temperature ,and G is the humidity constant of air. Letting P C be the thermal capacity with fixed pressure, λ the thermal conductivity ,and μ the dynamic viscosity of air flow, we calculate the thermal conductivity and kinematic viscosity using the formulas ρλP C =a and ρμν=. The thermal parameters of the surrounding rock are determined from the tunnel site.2 .3.2 Determination of the initial and boundary conditions .Choose the observed monthly average wind speed at the entry and exit as boundary conditions of wind speed ,and choose the relative effective pressure p=0 at the exit ( that is ,the entry of the dominant wind trend) and ]5[22/)/1(v d kL p ⨯+= on the section of entry ( that is ,the exit of the dominant wind trend ),where k is the coefficient of resistance along the tunnel wall, d = 2R ,and v is the axial average speed. We approximate T varying by the sine law according to the data observed at the scene and provide a suitable boundary value based on the position of the permafrost base and the geothermal gradient of the thaw rock materials beneath thepermafrost base.3 A simulated exampleUsing the model and the solving method mentioned above,we simulate the varying law of the air temperature in the tunnel along with the temperature at the entry and exit of the Xiluoqi No.2 Tunnel .We observe that the simulated results are close to the data observed[6].The Xiluoqi No .2 Tunnel is located on the Nongling railway in northeastern China and passes through the part beneath the permafrost base .It has a length of 1 160 m running from the northwest to the southeast, with the entry of the tunnel in the northwest,and the elevation is about 700 m. The dominant wind direction in the tunnel is from northwest to southeast, with a maximum monthly-average speed of 3 m/s and a minimum monthly-average speed of 1 .7 m/s . Based on the data observed,we approximate the varying sine law of air temperature at the entry and exit with yearly averages of -5℃,-6.4℃ and amplitudes of 18.9℃ and 17.6℃respectively. The equivalent diameter is 5 .8m,and the resistant coefficient along the tunnel wall is 0.025.Since the effect of the thermal parameter of the surrounding rock on the air flow is much smaller than that of wind speed,pressure and temperature at the entry and exit,we refer to the data observed in the Dabanshan Tunnel for the thermal parameters.Figure 1 shows the simulated yearly-average air temperature inside and at the entry and exit of the tunnel compared with the data observed .We observe that the difference is less than 0 .2 `C from the entry to exit.Figure 2 shows a comparison of the simulated and observed monthly-average air temperature in-side (distance greater than 100 m from the entry and exit) the tunnel. We observe that the principal law is almost the same,and the main reason for the difference is the errors that came from approximating the varying sine law at the entry and exit; especially , the maximum monthly-average air temperature of 1979 was not for July but for August.Fig.1. Comparison of simulated and observed air temperature in Xiluoqi No.2 Tunnel in 1979.1,simulated values;2,observed valuesFig.2.The comparison of simulated and observed air temperature inside The Xiluoqi No.2 Tunnel in 1979.1,simulated values;2,observed values4 Prediction of the freeze-thaw conditions for the Dabanshan Tunnel 4 .1 Thermal parameter and initial and boundary conditionsUsing the elevation of 3 800 m and the yearly-average air temperature of -3℃, we calculate the air density p=0 .774 kg/m 3.Since steam exists In the air, we choose the thermal capacity with a fixed pressure of air ),./(8744.10C kg kJ C p = heat conductivity )./(100.202C m W -⨯=λ andand the dynamic viscosity )../(10218.96s m kg -⨯=μ After calculation we obtain the thermal diffusivity a= 1 .3788s m /1025-⨯ and the kinematic viscosity ,s m /1019.125-⨯=ν .Considering that the section of automobiles is much smaller than that of the tunnel and the auto-mobiles pass through the tunnel at a low speed ,we ignore the piston effects ,coming from the movement of automobiles ,in the diffusion of the air.We consider the rock as a whole component and choose the dry volumetric cavity 3/2400m kg d =λ,content of water and unfrozen water W=3% and W=1%, and the thermal conductivity c m W o u ./9.1=λ,c m W o f ./0.2=λ,heatcapacityc kg kJ C o V ./8.0= and d u f W w C γ⨯++=1)128.48.0(,d u u Ww C γ⨯++=1)128.48.0( According to the data observed at the tunnel site ,the maximum monthly-average wind speed is about 3 .5 m/s ,and the minimum monthly-average wind speed is about 2 .5 m/s .We approximate the wind speed at the entry and exit as )/](5.2)7(028.0[)(2s m t t v +-⨯=, where t is in month. The initial wind speed in the tunnel is set to be.0),,0(),)(1(),,0(2=-=r x V Rr U r x U a The initial and boundary values of temperature T are set to bewhere f(x) is the distance from the vault to the permafrost base ,and R0=25 m is the radius of do-main of solution T. We assume that the geothermal gradient is 3%,the yearly-average air temperature outside tunnel the is A=-3C 0,and the amplitude is B=12C 0.As for the boundary of R=Ro ,we first solve the equations considering R=Ro as the first type of boundary; that is we assume that T=f(x)⨯3%C 0on R=Ro. We find that, after one year, the heat flow trend will have changed in the range of radius between 5 and 25m in the surrounding rock.. Considering that the rock will be cooler hereafter and it will be affected yet by geothermal heat, we appoximately assume that the boundary R=Ro is the second type of boundary; that is ,we assume that the gradient value ,obtained from the calculation up to the end of the first year after excavation under the first type of boundary value, is the gradient on R=Ro of T.Considering the surrounding rock to be cooler during the period of construction ,we calculatefrom January and iterate some elapses of time under the same boundary. Then we let the boundaryvalues vary and solve the equations step by step(it can be proved that the solution will not depend on the choice of initial values after many time elapses ).1)The yearly-average temperature on the surface wall of the tunnel is approximately equal to the ai4 .2 Calculated resultsFigures 3 and 4 show the variations of the monthly-average temperatures on the surface of the tunnel wall along with the variations at the entry and exit .Figs .5 and 6 show the year when permafrost begins to form and the maximum thawed depth after permafrost formed in different surrounding sections.Fig.3.The monthly-average temperature parison of the monthly- On the surface of Dabanshan Tunnel.I, average temperature on the surface The month,I=1,2,3,,,12 tunnel with that outside the tunnel. 1,inner temperature on the surface ;2,outside air temperatureFig.5.The year when permafrost Fig.6.The maximum thawed depth after Begins to from in different permafrost formed in different years Sections of the surroundingrock4 .3 Preliminary conclusionBased on the initial-boundary conditions and thermal parameters mentioned above, we obtain the following preliminary conclusions: r temperature at the entry and exit. It is warmer during the cold season and cooler during the warm season in the internal part (more than 100 m from the entry and exit) of the tunnel than at the entry and exit . Fig .1 shows that the internal monthly-average temperature on the surface of the tunnel wall is 1.2℃ higher in January, February and December, 1℃higher in March and October, and 1 .6℃ lower in June and August, and 2qC lower in July than the air temperature at the entry and exit. In other months the infernal temperature on the surface of the tunnel wall approximately equals the air temperature at the entry and exit.2) Since it is affected by the geothermal heat in the internal surrounding section,especially in the central part, the internal amplitude of the yearly-average temperature on the surface of the tunnel wall decreases and is 1 .6℃ lower than that at the entry and exit.3 ) Under the conditions that the surrounding rock is compact , without a great amount of under-ground water, and using a thermal insulating layer(as designed PU with depth of 0.05 m and heat conductivity λ=0.0216 W/m℃,FBT with depth of 0.085 m and heat conductivity λ=0.0517W/m℃),in the third year after tunnel construction,the surrounding rock will begin to form permafrost in the range of 200 m from the entry and exit .In the first and the second year after construction, the surrounding rock will begin to form permafrost in the range of 40 and 100m from the entry and exit respectively .In the central part,more than 200m from the entry and exit, permafrost will begin to form in the eighth year. Near the center of the tunnel,permafrost will appear in the 14-15th years. During the first and second years after permafrost formed,the maximum of annual thawed depth is large (especially in the central part of the surrounding rock section) and thereafter it decreases every year. The maximum of annual thawed depth will be stable until the 19-20th yearsand will remain in s range of 2-3 m.4) If permafrost forms entirely in the surrounding rock,the permafrost will provide a water-isolating layer and be favourable for communication and transportation .However, in the process of construction,we found a lot of underground water in some sections of the surrounding rock .It will permanently exist in those sections,seeping out water and resulting in freezing damage to the liner layer. Further work will be reported elsewhere.严寒地区隧道围岩冻融状况分析的导热与对流换热模型摘要通过对严寒地区隧道现场基本气象条件的分析,建立了隧道内空气与围岩对流换热及固体导热的综合模型;用此模型对大兴安岭西罗奇2号隧道的洞内气温分布进行了模拟计算,结果与实测值基本一致;分析预报了正在开凿的祁连山区大坂山隧道开通运营后洞内温度及围岩冻结、融化状况.关键词严寒地区隧道导热与对流换热冻结与融化在我国多年冻土分布及邻近地区,修筑了公路和铁路隧道几十座.由于隧道开通后洞内水热条件的变化;,普遍引起洞内围岩冻结,造成对衬砌层的冻胀破坏以及洞内渗水冻结成冰凌等,严重影响了正常交通.类似隧道冻害问题同样出现在其他国家(苏联、挪威、日本等)的寒冷地区.如何预测分析隧道开挖后围岩的冻结状况,为严寒地区隧道建设的设计、施工及维护提供依据,这是一个亟待解决的重要课题.在多年冻土及其临近地区修筑的隧道,多数除进出口部分外从多年冻土下限以下岩层穿过.隧道贯通后,围岩内原有的稳定热力学条件遭到破坏,代之以阻断热辐射、开放通风对流为特征的新的热力系统.隧道开通运营后,围岩的冻融特性将主要由流经洞内的气流的温度、速度、气—固交界面的换热以及地热梯度所确定.为分析预测隧道开通后围岩的冻融特性,Lu-nardini借用Shamsundar研究圆形制冷管周围土体冻融特性时所得的近似公式,讨论过围岩的冻融特性.我们也曾就壁面温度随气温周期性变化的情况,分析计算了隧道围岩的温度场[3].但实际情况下,围岩与气体的温度场相互作用,隧道内气体温度的变化规律无法预先知道,加之洞壁表面的换热系数在技术上很难测定,从而由气温的变化确定壁面温度的变化难以实现.本文通过气一固祸合的办法,把气体、固体的换热和导热作为整体来处理,从洞口气温、风速和空气湿度、压力及围岩的水热物理参数等基本数据出发,计算出围岩的温度场.1数学模型为确定合适的数学模型,须以现场的基本情况为依据.这里我们以青海祁连山区大坂山公路隧道的基本情况为背景来加以说明.大坂山隧道位于西宁一张业公路大河以南,海拔3754.78~3801.23 m ,全长1530 m ,隧道近西南—东北走向. 由于大坂山地区隧道施工现场平均气温为负温的时间每年约长8个月,加之施工时间持续数年,围岩在施土过程中己经预冷,所以隧道开通运营后,洞内气体流动的形态主要由进出口的主导风速所确定,而受洞内围岩地温与洞外气温的温度压差的影响较小;冬季祁连山区盛行西北风,气流将从隧道出曰流向进口端,夏季虽然祁连山区盛行东偏南风,但考虑到洞口两端气压差、温度压差以及进出口地形等因素,洞内气流仍将由出口北端流向进口端.另外,由于现场年平均风速不大,可以认为洞内气体将以层流为主基于以上基本情况,我们将隧道简化成圆筒,并认为气流、温度等关十隧道中心线轴对称,忽略气体温度的变化对其流速的影响,可有如下的方程:其中t 为时间,x 为轴向坐标,r 为径向坐标;U, V 分别为轴向和径向速度,T 为温度,P 为有效压力(即空气压力与空气密度之比少,V 为空气运动粘性系数,a 为空气的导温系数,L 为隧道长度,R 为隧道的当量半径,D 为时间长度)(t S f , )(t S u 分别为围岩的冻、融区域. f λ,u λ分别为冻、融状态下的热传导系数,f C ,u C 分别为冻、融状态下的体积热容量,X=(x,r) , )(t ξ为冻、融相变界面,To 为岩石冻结临界温度(这里具体计算时取To=-0.10C 0),h L 为水的相变潜热.2 求解过程由方程(1)知,围岩的温度的高低不影响气体的流动速度,所以我们可先解出速度,再解温度.2.1 连续性方程和动量方程的求解由于方程((1)的前3个方程不是相互独立的,通过将动量方程分别对x 和r 求导,经整理化简,我们得到关于压力P 的如下椭圆型方程:于是,对方程(1)中的连续性方程和动量方程的求解,我们按如下步骤进行:(1)设定速度0U ,0V ;( 2)将0U ,0V 代入方程并求解,得0P(3)联立方程(1)的第一个和第二个方程,解得一组解1U ,1V ;(4)联立方程((1)的第一个和第三个方程,解得一组解2U ,2V ;(5)对((3) ,(4)得到的速度进行动量平均,得新的0U ,0V 返回(2) ;(6)按上述方法进行迭代,直到前后两次的速度值之差足够小.以0P ,0U ,0V 作为本时段的解,下一时段求解时以此作为迭代初值.2. 2 能量方程的整体解法如前所述,围岩与空气的温度场相互作用,壁面既是气体温度场的边界,又是固体温度场的边界,壁面的温度值难以确定,我们无法分别独立地求解隧道内的气体温度场和围岩温度场.为克服这一困难,我们利用在洞壁表面上,固体温度等于气体温度这一事实,把隧道内气体的温度和围岩内固体的温度放在一起求解,这样壁面温度将作为末知量被解出来.只是需要注意两点:解流体温度场时不考虑相变和解固体温度时没有对流项;在洞壁表面上方程系数的光滑化.另外,带相变的温度场的算法与文献[3]相同.2. 3热参数及初边值的确定热参数的确定方法: 用p=1013.25-0.1088H 计算出海拔高度为H 的隧道现场的大气 压强,再由GT P =ρ计算出现场空气密度ρ,其中T 为现场大气的年平均绝对温度,G 为空气的气体常数.记定压比热为P C ,导热系数为λ,空气的动力粘性系数为μ.按ρλP C =a 和ρμν= 计算空气的导温系数和运动粘性系数.围岩的热物理参数则由现场采样测定.初边值的确定方法:洞曰风速取为现场观测的各月平均风速.取卞导风进曰的相对有效气压为0,主导风出口的气压则取为]5[22/)/1(v d kL p ⨯+=,这里k 为隧道内的沿程阻力系数,L 为隧道长度,d 为隧道端面的当量直径,ν为进口端面轴向平均速度.进出口气温年变化规律由现场观测资料,用正弦曲线拟合,围岩内计算区域的边界按现场多年冻土下限和地热梯度确定出适当的温度值或温度梯度. 3 计算实例按以上所述的模型及计算方法,我们对大兴安岭西罗奇2号隧道内气温随洞曰外气温变化的规律进行了模拟计算验证,所得结果与实测值[6]相比较,基本规律一致.西罗奇2号隧道是位十东北嫩林线的一座非多年冻土单线铁路隧道,全长1160 m ,隧道近西北一东南向,高洞口位于西北向,冬季隧道主导风向为西北风.洞口海拔高度约为700 m ,月平均最高风速约为3m/s,最低风速约为1.7m/s.根据现场观测资料,我们将进出口气温拟合为年平均分别为-5C 0和-6.4C 0,年变化振幅分别为18.9C 0和17.6C 0的正弦曲线.隧道的当量直径为5.8 m,沿程阻力系数取为0.025.由于围岩的热物理参数对计算洞内气温的影响远比洞口的风速、压力及气温的影响小得多,我们这里参考使用了大坂山隧道的资料.图1给出了洞口及洞内年平均气温的计算值与观测值比较的情况,从进口到出口,两值之差都小于0.2C 0.图2给出了洞内 (距进出口l00m 以上)月平均气温的计算值与观测值比较的情况,可以看出温度变化的基本规律完全一致,造成两值之差的主要原因是洞口气温年变化规律之正弦曲线的拟合误差,特别是1979年隧道现场月平均最高气温不是在7月份,而是在8月份.图1. 比较1979年在西罗奇周家山2号隧道仿真试验与观察的空气温度.1、模拟值;2、观测值图2。
路基沉降英文翻译

As follows:Reinforced geogrid can do its work only by enough soil structure interaction.With enlargement of interface element strength, engineering behavior changes of reinforced soil structure as follow: factor of safety enlarged; post-construction settlements, lateral displacement and consolidation time all decreased, the stress along the reinforced material tends to uniform and the max value decreased.If the strength of interface element is weak, effect of the interface element thickness change is not apparent; on the other hand, if that strength is strong the engineering behavior of reinforced soil structure increased with the interface element thickness.The major work of reinforced geogrid is at the nearby place, too many reinforced mate rial can’t develop the engineering behavior apparently.Reference(1)Yin Guang-zhi, ZHANG Dong-ming, and WEI Zuo-an. Testing study on inteaction characteristcs between fine grained tailings and geosynthetica.Chinese Journal of Rock Mechanics and Engineering,2003,23(3): 426-429 (2)Goodman, R E.Taylor, R L and Brekke, T L A model for the mechanics of joined rock. Soil Mech And Found. Engrg.Div.,ASCE,1968,94(3): 637-660 (3)Desai, C S. and Nagaraj, B K. Modeling for cyclic normal and shear behavior of interfaces. Engrg. Mech, ASCE. 1988.8(1):1198-1217(4)LU Ting-hao, BAO, Fu-bo. A coupled constitutive model for interface thin-layer element. Shuli Xuebao,2000, 2: 71-75(5)YIN Zong-ze, ZHU Hong, and XU Guo-hua. Nunserical simulation of deformation in the interface between soil and structural Yantu Gongcheng Xuebao, 1994, 16(3): 14-22(6)LV Peng, YANG Guang-qing. Research of interface behavior for safety of reinforced dam. Progress in Safety Science and Technology (2005 APSS) .Beijing: Science Press, 2005: 374-378(7)SONG Er-xiang. Finite element analysis of safety factor for soil structure.Chinese Journal of Geotechnique Engineering, 1997, 19(2): 1-7(8)Griffiths D.V, Lanc P. A. Slope stability analysis by finite element.Geotechnique. 1999, 49(3): 387-403Study on settlement of super highway roadbed ZHAO Yu, ZHANG Bao jian, LV peng, ZHOU QiaoyongEngineering department of Shijiazhuang railway institute,Shijiazhuang 050043, ChinaAbstract: in order to analyze the settlement rule of speedway roadbed, the test section of Qing-Ying speedway in Hebei province is selected to monitor the settlement rule of roadbed. The settling tube combined with test pile at the road shoulder is accepted to monitor the settlement rule and the teat data is get. According to the test results, this paper analyzes the settlement of the test section in Hebei province of Qing-Yin speedway. This paper also makes two-dimension model to analyze and forecast the rule by ADINA-a finite element software and concluded some distortion rule of the roadbed. By contrasting and analyzing the measured value and the calculated value, the conclusion is drawn. the result is that the most settlement comp completed in construction period and the settlement after completed is smaller and the settling rate becomes smaller, too. That is to say the increase settling value becomes smaller with the settlement increase. So the settlement after completed increase lies on the consolidation. But the most settlement completed at constructing, so the settling value most lies on the height of embankment. The settlement value increases with the height of embankment increases. So we should control the height of embankment to control the settlement of speedway roadbed.Key words: settlement; finite element; settle monitor1.IntroductionThe settlement and distortion study on speedway roadbed emphasize particularly on the intension destroy and control the settlement and distortion index. Before the intension destroy of roadbed occurred, the settlement and distortion impermissible of roadbed will occur at the speedway which take the intension as the first design index. This makes the roadbed crack or sink. The oversize or uneven distortion will reflect to the pavement and this will make the pavement settle and crack which has a strong impact on the transportation property. Longitudinal cracks which is a few km even over ten km occur at the Jing-Qin speedway Hebei section which completed at 1999. The similar questions also occur at the Cang-Huang speedway which completed at 2000. These questions impact the using strongly. All of questions show that taking the intension as the first design index is unreasonable and should take the settlement and distortion control as the first design index.The study focus on the soft soil bed and sock bed for taking intension as the firstdesign index of speedway and have a disregard of normal roadbed. This paper studies the settlement of normal roadbed at Qing-Yin speedway test section.The section in Hebei province of Qing-Yin spe edway is the component of “five portrait and seven landscape orientation” national main trunk line, is 183.551 km long. This section locates at the southwest of Huabei plain and at the fore proluvial fan of Taihang Mountain. The ground elevation is between 25m and 44m. this section is 450m long and the engineering geology is clayey soil, stiff plasticity and bearing capacity up to 120-200kPa and the side wall friction resistance up to 15-35kPa.The filling compactness demand sees the Table 1.In order to achieve the compactness, the loose laying depth should less than 30cm and filling should placement and ramming in layers.2.Settle monitorSelecting the 6.0m high test section at Qing-Yin speedway, and place the settlement gauging at the embank bottom to study the settlement rule during construction and after completed. The settlement gauging includes the digital display sliding type settlement gauging, settling tube, high response probe and the cable which joint to the gauging.In order to study the settlement rule of normal roadbed, placing the settling tube at the test section to control the settlement after completed and placing the test pile at the road shoulder when the embankment completed (see the Fig .1).The settling tube can measure the settling value of gross section. The purpose-made PVC tube is placed into the embank and draw the high response probe attached servo acceleration sensor form the one end to the other end of the tube. The sensor is at vengeance direction and the projection at sensing horizontal axis by the acceleration of gravity, which can measure the angle of the probe length. The two end height difference of probe accumulated to the settlement at the every point of the sub grade cross-section. The advantage of the settling gauging is ① high precision. The test verity the reading error should than 0.1mm every times and the accumulated error which testing 50m length should less than 5mm;②operation facility. The whole testing system (probe, cable and drawing wire, recording apparatus) can be carried by one people and move very convenience. So the testing system can operate only one people;③ the settlement curve of sub grade cross-section can be test by this gauging system.The value observation long to 363 days at this testing section before the embankment completed, the fore 63 day is the filling construction stage. The settlement after completed doesn’t acquire. This paper according to the FEM forecast to calculate the settlement after completed.3.FEM simulation and calculationThis paper takes the Mohr-coulomb to simulate and calculate the settlement and distortion of the roadbed. For the axial symmetry of embank and ground, the model is set up taking one second. The embankment and ground material is appointed as the elastic-perfectly plastic material which accords with Mohr-coulomb yield criterion and appoint ground as the porous medium.3.1 Constitutive relationThe elastoplasticity constitutive relation of soil mass is described as augmenter form. The augmenter method is to divide the whole load to some little augmenter and the calculation is carried with FEM by step. To calculate each augmenter, assuming the material properties doesn’t change and get the augmenter of strain, stress and displacement. The material properties and stiffness matrix change has reflected the nonlinear relative between stress and strain. This method actually is using the augmentation line to approach curve line.The element stress state different under the load increment {ΔRσ}action. When the element fall into the elastic range, the stiffness matrix should adopt the increment [D];when the element comes to plastic yield, the stiffness matrix must to use the plastic elasticity matrix [Dep]. Using the incremental method as the elastoplasticity calculate step:⑴ assume the material as the elastic material and use the elasticity matrix [D]to form [K], which is used to get the displace and to calculate the elastic stress increment. The final stress {σ*}={σ}B+{Δσ*}.⑵ using the yield rule to judge the stress variations is what, which is the key to adopt [D]or[Dep].⑶ when elastoplasticity finite element calculation is doing, the mid increment is adopted to calculate[D]or[Dep].3.2 Model dimension and key parameterThe half ground is 69m extension from the road center line. The embankment is 6m high and the upper surface width is 14m and the bottom width is 23m. The two dimension elastoplaticity model is 30cm every layer.The material parameters used in FEM calculate and analyzing are got from the laboratory test, which includes the compression test, shear test and permeability test. The main parameter is list in the Table 2.3.3 Construct step by step simulationThe load is the self-weight of embank. At normal stress deformation calculating, assume the embank fill to the top only once and the load is applied only once, too. So every part of the load is hold by the structure. Actually during load applying step by step, a certain height reached, which the filled embankment under is used to bear the load and the upper unfilled soil doesn’t take effect and the upper soil doesn’t affect the under soil. The construct realize by element death and birth in ADINA and according to the actual filling course to active the accordingly layer at the accordingly time and mesh the filled soil body only.4. Contrast settling process on-spot to the calculated settling processThe settlement date can be drawn by surveying the placed settling tube at the section.The settlement can be simulated and calculated by FEM and draw the settlement at the 0m, 4.5m, 9m, 16m and 23m day should be draw in the Fig.2. From the Fig.2 the settling curve on the spot and the calculated curve can meet very well at tendency, but the calculated value is less than the value measured on spot. The main cause for difference generating is that the model is set up by the normal foundation, but the consolidate rate of normal foundation is different to the actual foundation.In order to forecast the secular settlement of roadbed, this paper forecast the settlement at the 1563th day with FEM. From the Fig.3 the settling take lessen gradually and get to stabilization with the time increasing and the settling value after completed is less than the value at construct. Take the mid roadbed as example, thesettling value reach to 0.0938m from 0.063m at completion. From this, we can find that the settlement happen only during construct and the settling value after completed is very little. We also find that the tendence of the points at under surface is accordance and the settling value depends on the filling height-the load quantity. The filling depth is equal at the mid roadbed and the 16m from the tube end, that is to say the loads equal, so the settling value is almost equal. At the road shoulder (9m from the tube end), though the filling depth is equal to the mid road, the right side fill soil is decrease gradually, that is to the load is decrease gradually, so the settling value is less than the mid road. In Fig.4, the calculated settlement of point at the 1563th day is drawn. From Fig.3and Fig.4, we can find the settling tendence of point at the foundation surface, which meet with the actual curve.The convenient post treatment of ADINA offers kinds of cloud chart.Fig.5 is the settlement cloud chart at elevation direction at the 1563th day. From Fig.3, we can find the positive settlement at elevation direction is 0.6cm, which happen at almost 4m from slope toe and the negative settlement at elevation direction is 10.6cm,which happen at almost one third of embankment.。
双线地铁盾构施工引起的地表沉降分析及,施工控制研究
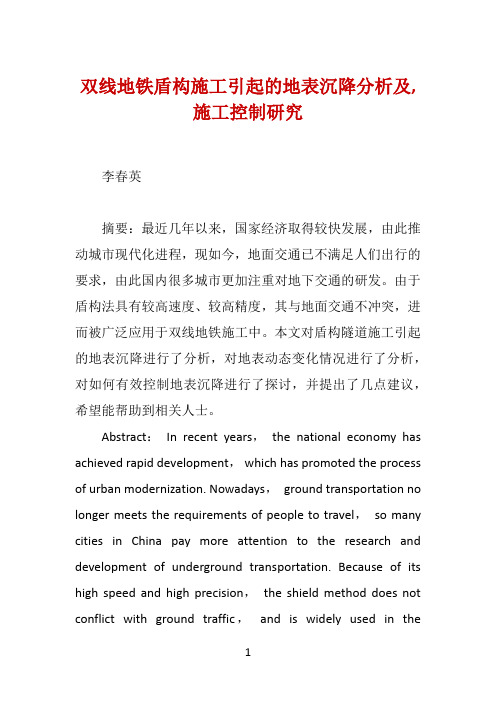
双线地铁盾构施工引起的地表沉降分析及,施工控制研究李春英摘要:最近几年以来,国家经济取得较快发展,由此推动城市现代化进程,现如今,地面交通已不满足人们出行的要求,由此国内很多城市更加注重对地下交通的研发。
由于盾构法具有较高速度、较高精度,其与地面交通不冲突,进而被广泛应用于双线地铁施工中。
本文对盾构隧道施工引起的地表沉降进行了分析,对地表动态变化情况进行了分析,对如何有效控制地表沉降进行了探讨,并提出了几点建议,希望能帮助到相关人士。
Abstract:In recent years,the national economy has achieved rapid development,which has promoted the process of urban modernization. Nowadays,ground transportation no longer meets the requirements of people to travel,so many cities in China pay more attention to the research and development of underground transportation. Because of its high speed and high precision,the shield method does not conflict with ground traffic,and is widely used in theconstruction of double-track subways. This paper analyzes the surface settlement caused by shield tunnel construction and the dynamic changes of the surface,discusses how to effectively control the surface settlement,and puts forward some suggestions,hoping to help relevant people.关键词:数值模型;盾构施工;地表动态;同步注浆Key words:numerical model;shield construction;surface dynamics;synchronous grouting中图分类号:P642.26 文獻标识码:A 文章编号:1006-4311(2020)20-0142-020 引言在地铁隧道施工中使用盾构法,会对施工周围的岩土产生影响,促使其发生振动,随着时间不断推移,会导致隧道上方地表发生沉降现象。
- 1、下载文档前请自行甄别文档内容的完整性,平台不提供额外的编辑、内容补充、找答案等附加服务。
- 2、"仅部分预览"的文档,不可在线预览部分如存在完整性等问题,可反馈申请退款(可完整预览的文档不适用该条件!)。
- 3、如文档侵犯您的权益,请联系客服反馈,我们会尽快为您处理(人工客服工作时间:9:00-18:30)。
外文原文Surface settlement predictions for Istanbul Metro tunnels excavated by EPB-TBMS. G. Ercelebi •H. Copur •I. OcakAbstract In this study, short-term surface settlements are predicted for twin tunnels, which are to be excavated in the chainage of 0 ? 850 to 0 ? 900 m between the Esenler and Kirazlıstations of the Istanbul Metro line, which is 4 km in length. The total length of the excavation line is 21.2 km between Esenler and Basaksehir. Tunnels are excavated by employing two earth pressure balance (EPB) tunnel boring machines (TBMs) that have twin tubes of 6.5 m diameter and with 14 m distance from center to center. The TBM in the right tube follows about 100 m behind the other tube. Segmental lining of 1.4 m length is currently employed as the final support. Settlement predictions are performed with finite element method by using Plaxis finite element program. Excavation, ground support and face support steps in FEM analyses are simulated as applied in the field. Predictions are performed for a typical geological zone, which is considered as critical in terms of surface settlement. Geology in the study area is composed of fill, very stiff clay, dense sand, very dense sand and hard clay, respectively, starting from the surface. In addition to finite element modeling, the surface settlements are also predicted by using semi-theoretical (semi-empirical) and analytical methods. The results indicate that the FE model predicts well the short-term surface settlements for a given volume loss value. The results of semi-theoretical and analytical methods are found to be in good agreement with the FE model. The results of predictions are compared and verified by field measurements. It is suggested that grouting of the excavation void should be performed as fast as possible after excavation of a section as a precaution against surface settlements during excavation. Face pressure of the TBMs should be closely monitored and adjusted for different zones.Keywords Surface settlement prediction _ Finite element method _ Analytical method _ Semi-theoretical method _ EPB-TBM tunneling _Istanbul MetroIntroductionIncreasing demand on infrastructures increases attention to shallow soft ground tunneling methods in urbanized areas. Many surface and sub-surface structures make underground construction works very delicate due to the influence of ground deformation, which should be definitely limited/controlled to acceptable levels. Independent of theexcavation method, the short- and long-term surface and sub-surface ground deformations should be predicted and remedial precautions against any damage to existing structures planned prior to construction. Tunneling cost substantially increases due to damages to structures resulting from surface settlements, which are above tolerable limits (Bilgin et al. 2009).Basic parameters affecting the ground deformations are ground conditions, technical/environmental parameters and tunneling or construction methods (O’Reilly and New 1982; Arioglu 1992; Karakus and Fowell 2003; Tan and Ranjit 2003; Minguez et al. 2005; Ellis 2005; Suwansawat and Einstein 2006). A thorough study of the ground by site investigations should be performed to find out the physical and mechanical properties of the ground and existence ofunderground water, as well as deformation characteristics, especially the stiffness. Technical parameters include tunnel depth and geometry, tunnel diameter–line–grade, single or double track lines and neighboring structures. The construction method, which should lead to a safe and economic project, is selected based on site characteristics and technical project constraints and should be planned so that the ground movements are limited to an acceptablelevel. Excavation method, face support pressure, advance (excavation) rate, stiffness of support system, excavation sequence and ground treatment/improvement have dramatic effects on the ground deformations occurring due to tunneling operations.The primary reason for ground movements above the tunnel, also known as surface settlements, is convergence of the ground into the tunnel after excavation, which changes the in situ stress state of the ground and results in stress relief. Convergence of the ground is also known as ground loss or volume loss. The volume of the settlement on the surface is usually assumed to be equal to the ground (volume) loss inside the tunnel (O’Reilly and New 1982). Ground loss can be classified as radial loss around the tunnel periphery and axial (face) loss at the excavation face (Attewell et al. 1986; Schmidt 1974). The exact ratio of radial and axial volume losses is not fully demonstrated or generalized in any study. However, it is possible to diminish or minimize the face loss in full-face mechanized excavations by applying a face pressure as a slurry of bentonite–water mixture or foam-processed muck. The ground loss is usually more in granular soils than in cohesive soils for similar construction conditions. The width of the settlement trough on both sides of the tunnel axis is wider in the case of cohesive soils, which means lower maximum settlement for the same amount of ground loss.Time dependency of ground behavior and existence of underground water distinguish short- and long-term settlements (Attewell et al. 1986). Short-term settlements occur during or after a few days (mostly a few weeks) of excavation, assuming that undrained soil conditions are dominant. Long-term settlements are mostly due to creep, stress redistribution and consolidation of soil after drainageof the underground water and elimination of pore water pressure inside the soil, and it may take a few months to a few years to reach a stabilized level. In dry soil conditions, the long-term settlements may be considered as very limited.There are mainly three settlement prediction approaches for mechanized tunnel excavations: (1) numerical analysis such as finite element method, (2) analytical method and (3) semi-theoretical (semi-empirical) method. Among them, the numerical approaches are the most reliable ones. However, the results of all methods should be used carefully by an experienced field engineer in designing the stage of an excavation project.In this study, all three prediction methods are employed for a critical zone to predict the short-term maximum surface settlements above the twin tunnels of the chainage between 0 ? 850 and 0 ? 900 m between Esenler and Kirazlı stations of Istanbul Metro line, which is 4 km in length. Plaxis finite element modeling program is used fornumerical modeling; the method suggested by Loganathan and Poulos (1998) is used for the analytical solution. A few different semi-theoretical models are also used for predictions. The results are compared and validated by field measurements.Description of the project, site and construction methodThe first construction phase of Istanbul Metro line was started in 1992 and opened to public in 2000. This line is being extended gradually, as well as new lines are being constructed in other locations. One of these metro lines is the twin line between Esenler and Basaksehir, which is 21.2 km. The excavation of this section has been started in May 2006. Currently, around 1,400 m of excavationhas already been completed. The region is highly populated including several story buildings, industrial zones and heavy traffic. Alignment and stations of the metro line between Esenler and Basaksehir is presented in Fig. 1.Totally four earth pressure balance (EPB) tunnel boring machines (TBM) are used for excavation of the tunnels. The metro lines in the study area are excavated by a Herrenknecht EPB-TBM in the right tube and a Lovat EPB-TBM in the left tube. Right tube excavation follows around 100 m behind the left tube. Some of the technical features of the machines are summarized in Table 1.Excavated material is removed by auger (screw conveyor) through the machine to a belt conveyor and than loaded to rail cars for transporting to the portal. Since the excavated ground bears water and includes stability problems, the excavation chamber is pressurized by 300 kPa and conditioned by applying water, foam, bentonite and polymers through the injection ports. Chamber pressure is continuously monitored by pressure sensors inside the chamber and auger. Installation of a segment ring with 1.4-m length (inner diameter of 5.7 m and outer diameter of 6.3 m) and 30-cm thickness is realized by a wing-type vacuum erector. The ring is configured as five segments plus a key segment. After installation of the ring, the excavation restarts and the void between the segment outer perimeter and excavated tunnel perimeter is grouted by300 kPa of pressure through the grout cannels in the trailing shield. This method of construction has been proven to minimize the surface settlements.The study area includes the twin tunnels of the chainage between 0 + 850 and 0 + 900 m, between Esenler and Kirazlı stations. Gungoren Formation of th e Miosen age is found in the study area. Laboratory and in situ tests are applied to define the geotechnical features of the formations that the tunnels pass through. The name, thickness and some of the geotechnical properties of the layers are summarized in Table 2 (Ayson 2005). Fill layer of 2.5-m thick consists of sand, clay, gravel and some pieces of masonry. The very stiff clay layer of 4 m is grayish green in color, consisting of gravel and sand. The dense sand layer of 5 m is brown at the upper levels and greenish yellow at the lower levels, consisting of clay, silt and mica. Dense sand of 3 m is greenish yellow and consists of mica. The base layer of the tunnel is hard clay, which is dark green, consisting of shell. The underground water table starts at 4.5 m below the surface. The tunnel axis is 14.5 m below the surface, close to the contact between very dense sand and hard clay. This depth isquite uniform in the chainage between 0 + 850 and 0 + 900 m.Surface settlement prediction with finite element modelingPlaxis finite element code for soil and rock analysis is used to predict the surface settlement. First, the right tube is constructed, and then the left tube 100 m behind the right tube is excavated. This is based on the assumption that ground deformations caused by the excavation of the right tube are stabilized before the excavation of the left tube. The finite element mesh is shown in Fig. 2 using 15 stress point triangular elements. The FEM model consists of 1,838 elements and 15,121 nodes. In FE modeling, the Mohr–Coulomb failure criterion is applied.Staged construction is used in the FE model. Excavation of the soil and the construction of the tunnel lining are carried out in different phases. In the first phase, the soil in front of TBM is excavated, and a support pressure of 300 kPa is applied at the tunnel face to prevent failure at the face. In the first phase, TBM is modeled as shell elements. In the second phase, the tunnel lining is constructedusing prefabricated concrete ring segments, which are bolted together within the tunnel boringmachine. During the erection of the lining, TBM remains stationary. Once a lining ring has been bolted, excavation is resumed until sufficient soil excavation is carried out for the next lining. The tunnel lining is modeled using volume elements. In the second phase, the lining is activated and TBM shell elements are deactivated.When applying finite element models, volume loss values are usually assumed prior to excavation. In this study, the FEM model is run with the assumption of 0.5, 0.75, 1 and 1.5% volume loss caused by the convergence of the ground into the tunnel after excavation. Figures 3 and 4 show total and vertical deformations after both tubes are constructed. The vertical ground settlement profile after theright tube construction is given in Fig. 5, which is in theshape of a Gaussian curve, and that after construction of both tubes is given in Fig. 6. Figure 7 shows the total deformation vectors.The maximum ground deformations under different volume loss assumptions are summarized in Table 3.Surface settlement prediction with semi-theoretical and analytical methodsSemi-theoretical predictions for short-term maximum settlement are performed using the Gaussian curve approach, which is a classical and conventional method. The settlement parameters used in semi-theoretical estimations and notations are presented in Fig. 8.The theoretical settlement (Gaussian) curve is presented as in Eq. 1 (O’Reilly and New 1982):)2(m a x 22i x e S S -= (1)where, S is the theoretical settlement (Gauss error function, normal probability curve), Smax is the maximum short-term (initial, undrained) settlement at the tunnel centerline (m), x is the transverse horizontal distance from the tunnel center line (m), and i is the point of inflexion (m). To determine the shape of a settlement curve, it is necessary to predict i and Smax values.There are several suggested methods for prediction of the point of inflexion (i). Estimation of i value in this studyis based on averages of some empirical approaches given in Eqs. 2–6:where, Z0 is the tunnel axis depth (m), 14.5 m in this study, and R is the radius of tunnel, 3.25 m in this study. Equation 3 was suggested by Glossop (O’Reilly and New 1982) for mostlycohesive grounds; Eq. 4 was suggested by O’Reilly and New (1982) for excavation of cohesive grounds by shielded machines; Eq. 5 was suggested by Schmidt (1969) for excavation of clays by shielded machines; Eq. 6 was suggested by Arioglu (1992) for excavation of all types of soils by shielded machines. As a result, the average i value is estimated to be 6.6 m in this study.There are several suggested empirical methods for the prediction of the maximum surface settlement (Smax).Schmidt suggested a model for the estimation of Smax value for a single tunnel in 1969 as given in Eq. 7 (through Arioglu 1992):where, K is the volume loss (%). Arioglu (1992), based on field data, found a good relationship between K and N (stability ratio) for face-pressurized TBM cases as in Eq. 8:where cn is the natural unit weight of the soil (kN/m3), the weighted averages for all the layers, which is 19 kN/m3 in this study; rS is the total surcharge pressure (kPa), assumed to be 20 kPa in this study; rT is TBM face pressure (kPa), which is 300 kPa in this study; and CU is the undrained cohesion of the soil (kPa), the weighted averages for all the layers, which is 50 kPa in this study assuming that CU is equal to SU (undrained shear strength of the soil). Allaverages are estimated up to very dense sand, excluding hard clay, since the tunnel axis passes around the contact between very dense sand and hard clay. The model yields 17.1 mm of initial maximum surface settlement.Herzog suggested a model for the estimation of Smax value in 1985 as given in Eq. 9 for a single tunnel and Eq. 10 for twin tunnels (through Arioglu 1992):where, E is the elasticity modulus of formation (kPa), the weighted averages for all the layers, which is 30,000 kPa in this study, and a is the distance between the tunnel axes, which is 14 m in this study. The model yields 49.9 and 58.7 mm of initial maximum surface settlements for the right and the left tube tunnel, which is 100 mm behind the right tube, respectively.There are several analytical models for the prediction of short-term maximum surface settlements for shielded tunneling operations (Lee et al. 1992; Loganathan and Poulos 1998; Chi et al. 2001; Chou and Bobet 2002; Park 2004). The method suggested by Loganathan and Poulos (1998) is used in this study. In this method, a theoretical gapparameter (g) is defined based on physical gap in the void, face losses and workmanship value, and then the gap parameter is incorporated to a closed form solution to predict elastoplastic ground deformations. The undrained gap parameter (g) is estimated by Eq. 12:where Gp is the physical gap representing the geometric clearance between the outer skin of the shield and the liner, is the thickness of the tail shield, d is the clearance required for erection of the liner, U*3D is the equivalent 3D elastoplastic deformation at the tunnel face, and w is a value that takes into account the quality of workmanship.Maximum short-term surface settlement is predicted by theoretical Eq. 13 (Loganathan and Poulos 1998):where, t is undrained Poisson’s ratio, assumed to be of maximum 0.5; g is the gap parameter (m), which is estimated to be 0.0128 m in this study; and x is transverse distance from the tunnel centerline (m) and it is assumed to be 0 m for the maximum surface settlement. The model yields 23.0 mm of undrained maximum surface settlement.Other parameters of settlement such as maximum slope, maximum curvature and so on are not mentioned in this study.Verification of predictions by field measurements and discussionThe results of measurements performed on the surface monitoring points, by Istanbul Metropolitan Municipality, are presented in Table 4 for the left and right tubes. As seen, the average maximum surface settlements are around 9.6 mm for the right tube and 14.4 mm for the left tube, which excavates 100 m behind the right tube. Themaximum surface settlements measured around 15.2 mm for the right tube and 26.3 mm for the left tube. Higher settlements are expected in the left tube since the previous TBM excavation activities on the right tube overlaps the previous deformation. The effect of the left tube excavation on deformations of the right tube is presented in Fig. 9. As seen, after Lovat TBM in the right tube excavates nearby the surface monitoring point 25, maximum surface settlement reaches at around 9 mm; however, while Herrenknecht TBM in the left tube passes the same point, maximum surface settlement reaches at around 29 mm (Fig. 10).If the construction method applied to the site is considered, long-term (consolidation) settlements are expected to be low, since the tail void is grouted immediately after excavation. The results of predictions mentioned above and observed maximum surface settlements are summarized in Table 5.The methods suggested by Loganathan and Poulos (1998) and Schmidt (1969) connected with Arioglu’s suggestion (1992) can predict the maximum short-term surface settlements only for a single tunnel. Plaxis finite element and Herzog (1985) models can predict deformations for twin tubes.Herzog’s model (1985) yields higher maximum surface settlements than the observed ones. The reason for that is that the database of the model includes both shielded tunnels and NATM (New Austrian Tunneling Method) tunnels, of which surface settlements are usually higher compared to shielded tunnels. Schmidt (1969), along withArioglu’s suggestion (1992), yields predictions close to observed.Plaxis finite element modeling gives the most realistic results, provided there is correct assumption of volume loss parameter, which is usually difficult to predict. The model provides simulation of excavation, lining, grouting and face pressure in a realistic manner to predict surface and sub-surface settlements. The volume loss parameter is usually assumed to be \1% for excavation with facepressure-balanced tunnel boring machines. The realized volume loss in the site is around 1% for this study.Currently, there is difficulty yet in modeling the deformation behavior of twin tunnels. One of the most impressive studies on this issue was performed by Chapman et al. (2004). However, Chapman’s semi-theoretical method still requires enlargement of the database to improve the suggested model in his paper.ConclusionsIn this study, three surface settlement prediction methods for mechanized twin tunnel excavations b etween Esenler and Kirazlı stations of Istanbul Metro Line are applied. Tunnels of 6.5-m diameters with 14-m distance between their centers are excavated by EPM tunnel boring machines. The geologic structure of the area can be classified as soft ground. Settlement predictions are performed by using FE modeling, and semi-theoretical (semi-empirical) and analytical methods. The measured results after tunneling are compared to predicted results. These indicate that the FE model predicts well the short time surface settlements for a given volume loss value. The results of some semi-theoretical and analytical methods are found to be in goodagreement with the FE model, whereas some methods overestimate the measured settlements. The FE model predicted the maximum surface settlement as 15.89 mm (1% volume loss) for the right tube, while the measured maximum settlement was 15.20 mm. For the left tube (opened after the right), FE prediction was 24.34 mm, while measured maximum settlement was 26.30 mm.中文翻译基于盾构法的Istanbul地铁施工引起的地面沉降预测摘要在这项研究中,研究的是双线隧道的短期地面沉降,选取线路里程总长为4km的Istanbul地铁从Esenler站到Kirazl站方向850到900m区间为研究对象。